Kidney: Structure, Function and Related Diseases
The structure of human kidney can be seen as two reddish bean-shaped organs that are located below the rib cage on each side of the spine. They are almost a fistful in size, measuring around 10-12cm. Kidneys are the main organs in the human excretory system, which takes part in the filtration of the blood before the urine is formed. Let us look at the structure of the organ for a better understanding.
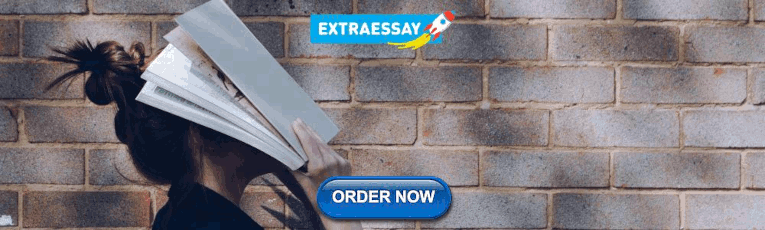
External and Internal Features of Kidney
- It has a convex and concave border.
- Towards the inner concave side, a notch called the hilum is present through which the renal artery enters the kidney and the renal vein and ureter leave.
- The outer layer of the kidney is a tough capsule.
- On the inside, the kidney is divided into an outer renal cortex and an inner renal medulla.
- The hilum extends inside the kidney into a funnel-like space called the renal pelvis.
- The renal pelvis has projections called calyces(sing: calyx).
- The medulla is divided into medullary pyramids, which project into the calyces.
- Between the medullary pyramids, the cortex extends as renal columns called Columns of Bertini.
- The kidney is made up of millions of smaller units called nephrons which are also the functional units.
Read: Nephrons
A simple structure of kidney can be understood by the following diagram:
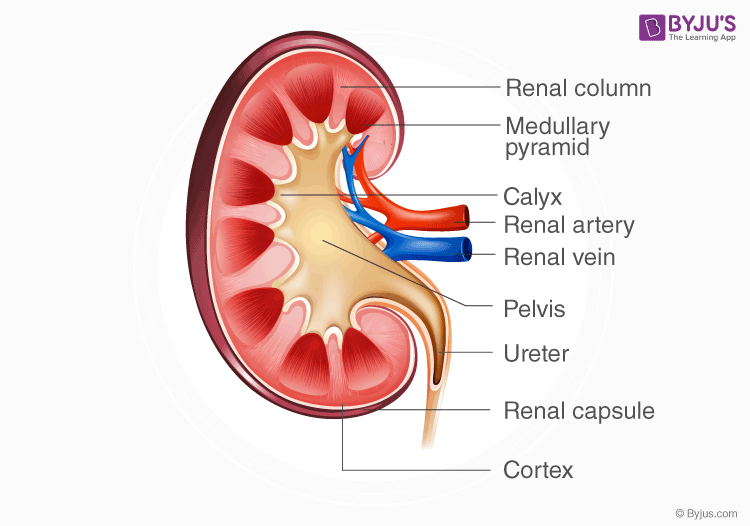
- The most important function of the kidney is to filter the blood for urine formation.
- It excretes metabolic wastes like urea and uric into the urine.
- Erythropoietin: It is released in response to hypoxia
- Renin: It controls blood pressure by regulation of angiotensin and aldosterone
- Calcitriol: It helps in the absorption of calcium in the intestines
- It maintains the acid-base balance of the body by reabsorbing bicarbonate from urine and excreting hydrogen ions and acid ions into the urine.
- It also maintains the water and salt levels of the body by working together with the pituitary gland .
Read: Urine Formation
Diseases related to Kidneys
In uremia, the kidneys are damaged, and there is a buildup of urea and other toxins in the blood, which is fatal and can cause kidney failure. Patients may experience fatigue, itching, muscle twitching, and loss of mental concentration. The urea can be removed by the process of hemodialysis.
2. Renal Calculi
Commonly called kidney stones, these are deposits of salt and minerals in our body. Symptoms include severe abdominal pain and nausea. The stones can be dissolved with medicines, or it passes with urine by improving diet and water intake.
3. Glomerulonephritis
It is the inflammation of the glomerulus. Symptoms include pink urine, oedema or swelling on the face and high blood pressure. It requires medical attention for prevention.
Note: Any person with high blood pressure and diabetes is more prone to kidney related diseases.
Explore BYJU’S Biology to learn more.
- Facts about Kidneys
- Regulation Of Kidney Function
- Kidney Function Test
- Kidney Failure Symptoms
- Difference Between Left and Right Kidney
What are the first signs of kidney problems?
You can see blood in your urine and it is also foamy. You will have a problem concentrating on things and will face swelling and dryness on your skin.
Can you live without a kidney?
It is not possible to live without a kidney. But since we have two of them, it is possible to survive with one kidney.
What causes kidney problems?
People with diseases like diabetes and high blood pressure have a high chance of developing kidney problems.
Leave a Comment Cancel reply
Your Mobile number and Email id will not be published. Required fields are marked *
Request OTP on Voice Call
Post My Comment

Thanking you Team Biju’s for this knowledge free of cost 😊

Register with BYJU'S & Download Free PDFs
Register with byju's & watch live videos.
The Normal Anatomy and Physiology of the Kidneys: Urine Formation Essay
The external anatomy of kidney, the internal anatomy of kidney, physiological balancing in the nephron.
Kidneys are the major organs of the renal system which perform vital homeostatic processes such as maintenance of water and ionic balance in the body. The kidneys’ primary function is waste removal through ultrafiltration, leading to urine formation. Moreover, they are actively involved in the reabsorption of amino acids, glucose, and water to achieve osmotic balance in the body (Wingerd & Taylor, 2020). In addition, hormones and enzymes are produced from the kidneys, stimulating, and catalysing physiological reactions in the body to achieve homeostasis.
A normal kidney is a brown organ, which has the shape of a bean seed. Each kidney is protected by a cushion called the renal capsule, a fibrous membrane having an irregular network of connecting tissue. The capsule is essential in holding kidneys in their positions within the abdominal cavity (Wingerd & Taylor, 2020). Moreover, it assists in maintaining the shape and responsible for protecting them from mechanical shock. The capsule is also overlayed by the renal fat pad, enhancing its efficacy in reducing the physical impact on the kidneys from an external force. The adipose tissues forming the fat pad are linked to the renal fascia, which in conjunction with the peritoneum, serves as anchorage surfaces for the kidneys to the posterior side of the abdominal cavity (Hickling et al., 2017). The hilum forms the entry point through which renal arteries and veins, and ureters serve the kidneys with fluids flowing in and out.
On top of the kidney is embedded an adrenal gland, which is responsible for modulating the organ’s physiological functions. However, the adrenal glands are part of the endocrine and not the renal system. The anterior interests of kidneys are protected by ribcages that curve into the lumbar region (Hickling et al., 2017). Kidneys are served by blood from the renal artery, which branches from the posterior side of the aorta. The flowing blood from the kidney is channelled into the inferior vena cava through the renal vein (Wingerd & Taylor, 2020). The excretory waste constituting the urine flows from the kidney into the bladder through the ureter. Thus, kidney serves in the purification of blood during the process of urine formation.
The kidney comprises three primary internal layers: the cortex, medulla, and pelvis. The renal cortex is the region immediately after the capsule, and within it are the extended sections of the nephron to form the Bowman’s capsule (Lawrence et al., 2018). It is a granular tissue within the kidney, offering a space for the arterioles and venules emerging from the arteries and veins, respectively. Moreover, the glomerular capillaries, which permit filtration of the blood components, are embedded in the cortex (Wingerd & Taylor, 2020). It is in the renal cortex where erythropoietin hormone is secreted to stimulate erythrocyte formation.
The medulla is the parenchymatous region within the kidney, constituting the immediate layer after the cortex. It is composed of stacked masses of tissue defined as renal pyramids. Each pyramid is comprised of densely interwoven nephrons (Wingerd & Taylor, 2020). The basic unit by which a kidney executes homeostatic function is the nephron. The Bowman capsule located in the cortex connects to the proximal convoluted tubules through which the glomerular filtered plasma flows (Hickling et al., 2017). In the medulla pyramids, the loop of Henle and the distal convoluted tubules form part of the nephron channelling the purified plasma into the collecting ducts.
The renal pelvis is the innermost concaved region of the kidney. Every pyramid of the medulla ends in a renal papilla that supplies concentrated urine into the minor calyces. The pool formed by minor calyces from every renal pyramid constitutes the major calyx. All the significant calyces are consecrated into a single unit called the pelvis (Wingerd & Taylor, 2020). The pelvis connects the kidney to the ureter, thus directing the concentrated urine into the bladder.
The Blood Supply Network
Kidneys are highly vascularized organs, receiving a quarter of the blood circulating within the body at a specific duration of time. The entry of blood into the two kidneys occurs via a pair of renal arteries extending from the aorta. On reaching the hilum of each kidney, the blood is channelled into segmental arteries, which branch into the interlobar arteries (Hickling et al., 2017). The interlobar vessels pass through the columns get into the cortex, in which they branch to form arcuate arteries. Further branching of the blood vessels leads to cortical radiate arteries, which supply their contents into the arterioles. Blood from the arterioles enters the glomerulus via the afferent arteriole. After glomerular filtration, the blood leaves the network of capillaries via the efferent arteriole (McDonald, 2019). Moreover, a portal of blood vessels extends from the afferent and efferent arterioles surrounding the proximal convoluted tubules, the loop of Henle, and the distal convoluted tubule to aid the urine concentration process.
Ultrafiltration in the Glomerulus
The formation of urine begins with the filtration process, which takes place in the glomerulus, a mesh of capillaries connected to the Bowman’s capsule. Ultrafiltration in the glomerulus does not require energy. However, it is accomplished through pressure build-up, which pushes the plasma and solute particles through the capillary walls. The process of filtration is aided through a three-layered membrane system (Lawrence et al., 2018). The fenestrated endothelia of capillaries in the permits plasma to pass through them, and not blood cells. Immediately, the negatively charged basement membrane blocks proteins from passing. Finally, the capsule of the capsule in the glomeruli develops a barrier that allows for the selected particles’ filtration. The efficiency of the filtration process is determined by the pressure create by the cardiac pumps of blood through the aorta, arteries, arterioles, and capillaries (Hickling et al., 2017). The net force for filtration generated in the glomeruli yields the glomerular filtrate channelled into the proximal convoluted tubule via the Bowman’s capsule.
Urine Concentration through Water and Ion Re-absorption
The kidney nephron is characterized by four tubular components in which reabsorption of water, ions, amino acids, and glucose takes place. The proximal convoluted tubule is attributed to the highest capacity of absorbing elements of the glomerular filtrate. From its lumen, sodium ions are taken back to the bloodstream by an active transport mechanism involving basolateral pumping of sodium-potassium ions (Lawrence et al., 2018). The secondary dynamic transport mechanism is involved in the reabsorption of amino acids, glucose, and vitamins. Moreover, water is reabsorbed by osmosis created by the ionic imbalance, which in turn drives the diffusion of lipids across the wall of proximal convoluted tubule into the bloodstream (Gupta & Sharma, 2020). The reabsorption of ions, sugar, amino acids, and lipids is essential in osmoregulation all over the body.
The glomerular filtrate moves into the loop of Henle, having the ascending and descending sections. The reabsorption of water through osmosis into the bloodstream main occurs in the descending spiral. On the other hand, potassium, sodium, and chloride ions are taken back to the bloodstream via the ascending loop of Henle (McDonald, 2019). The ATPase enzyme drives the process by creating an ionic gradient which makes the basolateral membrane of the symporter in the ascending loop to be functional in absorbing ions. Immediately after the loop of Henle, the filtrate enters the distal convoluted tubule where sodium ion absorption occurs. Mainly, active transport is involved in sodium-ion uptake via basolateral membrane (Lawrence et al., 2018). However, its passive absorption into the bloodstream occurs through sodium and chloride ion symporter on the apical plasmalemma. In the distal convoluted tubule, aldosterone hormone regulates sodium ion intake while parathyroid hormone controls the reabsorption of calcium ion (McDonald, 2019). Eventually, concentrated urine remaining in the lumen of the tubules moves into the collecting ducts, where final reabsorption occurs via active transport.
The concentrated urine is channelled into the pelvis; after that, it travels to the urinary bladder via the ureter. The kidney has two major homeostatic roles in the body, that is, maintenance of pH through balancing hydrogen ion concentration and osmoregulation. Moreover, it aids the purification of the blood by removing excess water, salts, and impurities. Its physiological functions encompass energy and the regulated hormonal process leading to the reabsorption of ions into the bloodstream. Thus, the structural and physiological function of the kidney allows urine formation and purification of blood.
Gupta, R., & Sharma, T. (2020). Review of urine formation in Ayurveda. Journal of Ayurveda and Integrated Medical Sciences, 5 (1), 145-148.
Hickling, D. R., Sun, T. T., & Wu, X. R. (2017). Anatomy and physiology of the urinary tract: relation to host defence and microbial infection . In Urinary Tract Infections: Molecular Pathogenesis and Clinical Management (pp. 1-25).
Lawrence, E. A., Doherty, D., & Dhanda, R. (2018). Function of the nephron and the formation of urine . Anaesthesia and Intensive Care Medicine, 19 (5), 249-253.
McDonald, M. D. (2019). The renal contribution to salt and water balance. In Fish Osmoregulation (pp. 309-331). CRC Press.
Wingerd, B., & Taylor, T. B. (2020). The Human Body: Concepts of Anatomy and Physiology . Jones & Bartlett Learning.
- The Mechanisms of Kidney Function
- Pathophysiologic Alterations of the Renal and Urologic Systems
- Kidney Function Tests: Chemical Methods Used to Diagnose Kidney Disease
- Lumbar Disk Herniation: Medical Analysis
- Intracranial Pressure Anatomy
- Physiotherapy and Fractured Neck of Femur
- Motor Skills Development of Young Children
- Rheumatoid Arthritis: Causes, Symptoms, Treatments
- Chicago (A-D)
- Chicago (N-B)
IvyPanda. (2021, October 21). The Normal Anatomy and Physiology of the Kidneys: Urine Formation. https://ivypanda.com/essays/the-normal-anatomy-and-physiology-of-the-kidneys-urine-formation/
"The Normal Anatomy and Physiology of the Kidneys: Urine Formation." IvyPanda , 21 Oct. 2021, ivypanda.com/essays/the-normal-anatomy-and-physiology-of-the-kidneys-urine-formation/.
IvyPanda . (2021) 'The Normal Anatomy and Physiology of the Kidneys: Urine Formation'. 21 October.
IvyPanda . 2021. "The Normal Anatomy and Physiology of the Kidneys: Urine Formation." October 21, 2021. https://ivypanda.com/essays/the-normal-anatomy-and-physiology-of-the-kidneys-urine-formation/.
1. IvyPanda . "The Normal Anatomy and Physiology of the Kidneys: Urine Formation." October 21, 2021. https://ivypanda.com/essays/the-normal-anatomy-and-physiology-of-the-kidneys-urine-formation/.
Bibliography
IvyPanda . "The Normal Anatomy and Physiology of the Kidneys: Urine Formation." October 21, 2021. https://ivypanda.com/essays/the-normal-anatomy-and-physiology-of-the-kidneys-urine-formation/.
Thank you for visiting nature.com. You are using a browser version with limited support for CSS. To obtain the best experience, we recommend you use a more up to date browser (or turn off compatibility mode in Internet Explorer). In the meantime, to ensure continued support, we are displaying the site without styles and JavaScript.
- View all journals
- Explore content
- About the journal
- Publish with us
- Sign up for alerts
- Review Article
- Published: 28 August 2012
The role of the kidney in regulating arterial blood pressure
- Hani M. Wadei 1 &
- Stephen C. Textor 2
Nature Reviews Nephrology volume 8 , pages 602–609 ( 2012 ) Cite this article
97 Citations
12 Altmetric
Metrics details
- Hypertension
The kidney plays a central role in the regulation of arterial blood pressure. A large body of experimental and physiological evidence indicates that renal control of extracellular volume and renal perfusion pressure are closely involved in maintaining the arterial circulation and blood pressure. Renal artery perfusion pressure directly regulates sodium excretion—a process known as pressure natriuresis—and influences the activity of various vasoactive systems such as the renin–angiotensin–aldosterone system. As a result, many researchers argue that identifying any marked rise in blood pressure requires resetting of the relationship between arterial blood pressure and urinary sodium excretion, which can occur by an array of systemic or local mechanisms. Almost all of the monogenic forms of hypertension affect sites in the kidney associated with sodium handling and transport. Experimental models of spontaneous hypertension, such as the Dahl salt-sensitive rat, have been used to study the effects of kidney transplantation on blood pressure. Results from studies of kidney transplantation indicate that pressure sensitivity to sodium intake 'follows' the kidney, meaning that the recipient of a 'salt-resistant kidney' acquires sodium resistance, and that the recipient of a 'salt-sensitive kidney' acquires pressure sensitivity. The examples above and discussed in this Review demonstrate that it should come as no surprise that most disorders that affect the kidney or the renal vasculature commonly lead to secondary forms of hypertension.
Renal artery perfusion pressure directly regulates sodium excretion, a process known as pressure natriuresis
The renin–angiotensin–aldosterone system has a central role in maintaining the pressure–natriuresis relationship
Maladaptive changes in tubular sodium and chloride handling leads to arterial hypertension despite the presence of a normal glomerular filtration rate
Rare inherited forms of hypertension involve gain or loss of function mutations in a single gene and are associated with increased sodium reabsorption in the distal nephron
Reduced nephron number contributes to the development of hypertension
The kidney is the origin of afferent sympathetic signalling that modulates the sympathetic nervous system, the activity of which increases in patients with renal failure
This is a preview of subscription content, access via your institution
Access options
Subscribe to this journal
Receive 12 print issues and online access
195,33 € per year
only 16,28 € per issue
Buy this article
- Purchase on Springer Link
- Instant access to full article PDF
Prices may be subject to local taxes which are calculated during checkout
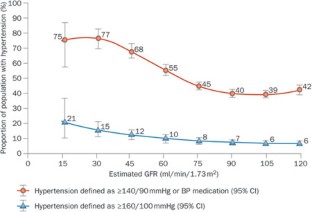
Similar content being viewed by others
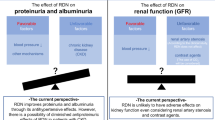
Effects of renal denervation on the kidney: albuminuria, proteinuria, and renal function
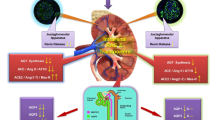
The intrarenal blood pressure modulation system is differentially altered after renal denervation guided by different intensities of blood pressure responses
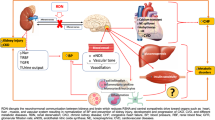
Possible organ-protective effects of renal denervation: insights from basic studies
Kearney, P. M. et al . Global burden of hypertension: analysis of worldwide data. Lancet 365 , 217–223 (2005).
Article PubMed Google Scholar
Udani, S., Lazich, I. & Bakris, G. L. Epidemiology of hypertensive kidney disease. Nat. Rev. Nephrol. 7 , 11–21 (2011).
US Renal Data System. 2011 Annual Data Report [online] , (2011).
Josephson, S., Lännergren, K. & Eklöf, A. C. Partial ureteric obstruction in weanling rats. II. Long-term effects on renal function and arterial blood pressure. Urol. Int. 48 , 384–390 (1992).
Article CAS PubMed Google Scholar
Bianchi, G., Fox, U., Di Francesco, G. F., Giovanetti, A. M. & Pagetti, D. Blood pressure changes produced by kidney cross-transplantation between spontaneously hypertensive rats and normotensive rats. Clin. Sci. Mol. Med. 47 , 435–448 (1974).
CAS PubMed Google Scholar
Patschan, O., Kuttler, B., Heemann, U., Uber, A. & Rettig, R. Kidneys from normotensive donors lower blood pressure in young transplanted spontaneously hypertensive rats. Am. J. Physiol. 273 , R175–R180 (1997).
Guidi, E. et al . Hypertension may be transplanted with the kidney in humans: a long-term historical prospective follow-up of recipients grafted with kidneys coming from donors with or without hypertension in their families. J. Am. Soc. Nephrol. 7 , 1131–1138 (1996).
Chun, T. Y. et al . Ethnic differences in renal responses to furosemide. Hypertension 52 , 241–248 (2008).
Guerrot, D. et al . Reduced insulin secretion and nocturnal dipping of blood pressure are associated with a disturbed circadian pattern of urine excretion in metabolic syndrome. J. Clin. Endocrinol. Metab. 96 , E929–E933 (2011).
Burnier, M. Alterations of renal sodium handling in arterial hypertension. Curr. Hypertens. Rep. 10 , 85–86 (2008).
Sinclair, A. M. et al . Secondary hypertension in a blood pressure clinic. Arch. Intern. Med. 147 , 1289–1293 (1987).
National Kidney Foundation. K/DOQI clinical practice guidelines for chronic kidney disease: evaluation, classification, and stratification. Am. J. Kidney Dis. 39 (Suppl. 1), S1–S266 (2002).
Sarafidis, P. A. et al . Hypertension awareness, treatment, and control in chronic kidney disease. Am. J. Med. 121 , 332–340 (2008).
Plantinga, L. C. et al . Blood pressure control among persons without and with chronic kidney disease: US trends and risk factors 1999–2006. Hypertension 54 , 47–56 (2009).
Guyton, A. C. Renal function curves and control of body fluids and arterial pressure. Acta Physiol. Scand. Suppl. 591 , 107–113 (1990).
Guyton, A. C. Physiologic regulation of arterial pressure. Am. J. Cardiol. 8 , 401–407 (1961).
Wang, C. T., Chin, S. Y. & Navar, L. G. Impairment of pressure-natriuresis and renal autoregulation in ANG II-infused hypertensive rats. Am. J. Physiol. Renal Physiol. 279 , F319–F325 (2000).
Suckling, R. J., He, F. J., Markandu, N. D. & MacGregor, G. A. Dietary salt influences postprandial plasma sodium concentration and systolic blood pressure. Kidney Int. 81 , 407–411 (2012).
Kawasaki, T., Delea, C. S., Bartter, F. C. & Smith, H. The effect of high-sodium and low-sodium intakes on blood pressure and other related variables in human subjects with idiopathic hypertension. Am. J. Med. 64 , 193–198 (1978).
Weinberger, M. H. Sodium sensitivity of blood pressure. Curr. Opin. Nephrol. Hypertens. 2 , 935–939 (1993).
Weinberger, M. H., Miller, J. Z., Luft, F. C., Grim, C. E. & Fineberg, N. S. Definitions and characteristics of sodium sensitivity and blood pressure resistance. Hypertension 8 , II127–II134 (1986).
Chiolero, A., Maillard, M., Nussberger, J., Brunner, H. R. & Burnier, M. Proximal sodium reabsorption: an independent determinant of blood pressure response to salt. Hypertension 36 , 631–637 (2000).
Fujii, T. et al . Circadian rhythm of natriuresis is disturbed in nondipper type of essential hypertension. Am. J. Kidney Dis. 33 , 29–35 (1999).
Uzu, T. et al . High sodium sensitivity implicates nocturnal hypertension in essential hypertension. Hypertension 28 , 139–142 (1996).
Fuenmayor, N., Moreira, E. & Cubeddu, L. X. Salt sensitivity is associated with insulin resistance in essential hypertension. Am. J. Hypertens. 11 , 397–402 (1998).
Galletti, F. et al . NaCl sensitivity of essential hypertensive patients is related to insulin resistance. J. Hypertens. 15 , 1485–1491 (1997).
Yatabe, M. S. et al . Salt sensitivity is associated with insulin resistance, sympathetic overactivity, and decreased suppression of circulating renin activity in lean patients with essential hypertension. Am. J. Clin. Nutr. 92 , 77–82 (2010).
Bigazzi, R. et al . Microalbuminuria in salt-sensitive patients. A marker for renal and cardiovascular risk factors. Hypertension 23 , 195–199 (1994).
de la Sierra, A. et al . Increased left ventricular mass in salt-sensitive hypertensive patients. J. Hum. Hypertens. 10 , 795–799 (1996).
Biollaz, J., Waeber, B., Diezi, J., Burnier, M. & Brunner, H. R. Lithium infusion to study sodium handling in unanesthetized hypertensive rats. Hypertension 8 , 117–121 (1986).
Burnier, M., Biollaz, J., Magnin, J. L., Bidlingmeyer, M. & Brunner, H. R. Renal sodium handling in patients with untreated hypertension and white coat hypertension. Hypertension 23 , 496–502 (1994).
Barba, G. et al . Renal function and blood pressure response to dietary salt restriction in normotensive men. Hypertension 27 , 1160–1164 (1996).
Machnik, A. et al . Macrophages regulate salt-dependent volume and blood pressure by a vascular endothelial growth factor-C-dependent buffering mechanism. Nat. Med. 15 , 545–552 (2009).
Burnier, M. Sodium balance and hypertension: rare genetic disorders expose pathogenic mechanisms. Exp. Nephrol. 6 , 496–501 (1998).
Chiolero, A., Würzner, G. & Burnier, M. Renal determinants of the salt sensitivity of blood pressure. Nephrol. Dial. Transplant. 16 , 452–458 (2001).
Boer, P. A., Morelli, J. M., Figueiredo, J. F. & Gontijo, J. A. Early altered renal sodium handling determined by lithium clearance in spontaneously hypertensive rats (SHR): role of renal nerves. Life Sci. 76 , 1805–1815 (2005).
Cusi, D. et al . Polymorphisms of α-adducin and salt sensitivity in patients with essential hypertension. Lancet 349 , 1353–1357 (1997).
Tripodi, G. et al . Hypertension-associated point mutations in the adducin alpha and beta subunits affect actin cytoskeleton and ion transport. J. Clin. Invest. 97 , 2815–2822 (1996).
Article CAS PubMed PubMed Central Google Scholar
Poch, E. et al . Molecular basis of salt sensitivity in human hypertension. Evaluation of renin-angiotensin-aldosterone system gene polymorphisms. Hypertension 38 , 1204–1209 (2001).
Kidambi, S. et al . Non-replication study of a genome-wide association study for hypertension and blood pressure in African Americans. BMC Med. Genet. 13 , 27 (2012).
Toka, H. R. & Luft, F. C. Monogenic forms of human hypertension. Semin. Nephrol. 22 , 81–88 (2002).
Ji, W. et al . Rare independent mutations in renal salt handling genes contribute to blood pressure variation. Nat. Genet. 40 , 592–599 (2008).
Wilson, F. H. et al . Molecular pathogenesis of inherited hypertension with hyperkalemia: the Na-Cl cotransporter is inhibited by wild-type but not mutant WNK4. Proc. Natl Acad. Sci. USA 100 , 680–684 (2003).
Lifton, R. P. et al . Hereditary hypertension caused by chimaeric gene duplications and ectopic expression of aldosterone synthase. Nat. Genet. 2 , 66–74 (1992).
Ritz, E., Amann, K., Koleganova, N. & Benz, K. Prenatal programming—effects on blood pressure and renal function. Nat. Rev. Nephrol. 7 , 137–144 (2011).
Hoy, W. E., Hughson, M. D., Bertram, J. F., Douglas-Denton, R. & Amann, K. Nephron number, hypertension, renal disease, and renal failure. J. Am. Soc. Nephrol. 16 , 2557–2564 (2005).
Hughson, M. D., Douglas-Denton, R., Bertram, J. F. & Hoy, W. E. Hypertension, glomerular number, and birth weight in African Americans and white subjects in the southeastern United States. Kidney Int. 69 , 671–678 (2006).
Nyengaard, J. R. & Bendtsen, T. F. Glomerular number and size in relation to age, kidney weight, and body surface in normal man. Anat. Rec. 232 , 194–201 (1992).
Brenner, B. M. & Chertow, G. M. Congenital oligonephropathy and the etiology of adult hypertension and progressive renal injury. Am. J. Kidney Dis. 23 , 171–175 (1994).
Hoy, W. E., Hughson, M. D., Singh, G. R., Douglas-Denton, R. & Bertram, J. F. Reduced nephron number and glomerulomegaly in Australian Aborigines: a group at high risk for renal disease and hypertension. Kidney Int. 70 , 104–110 (2006).
Keller, G., Zimmer, G., Mall, G., Ritz, E. & Amann, K. Nephron number in patients with primary hypertension. N. Engl. J. Med. 348 , 101–108 (2003).
Zimanyi, M. A. et al . Nephron number and individual glomerular volumes in male Caucasian and African American subjects. Nephrol. Dial. Transplant. 24 , 2428–2433 (2009).
Article PubMed PubMed Central Google Scholar
Rule, A. D. et al . Association of kidney function and metabolic risk factors with density of glomeruli on renal biopsy samples from living donors. Mayo Clin. Proc. 86 , 282–290 (2011).
Textor, S. C. et al . Association of filtered sodium load with medullary volumes and medullary hypoxia in hypertensive African Americans as compared with whites. Am. J. Kidney Dis. 59 , 229–237 (2012).
Aviv, A., Hollenberg, N. K. & Weder, A. B. Sodium glomerulopathy: tubuloglomerular feedback and renal injury in African Americans. Kidney Int. 65 , 361–368 (2004).
Genovese, G. et al . Association of trypanolytic ApoL1 variants with kidney disease in African Americans. Science 329 , 841–845 (2010).
Esler, M., Lambert, E. & Schlaich, M. Point: Chronic activation of the sympathetic nervous system is the dominant contributor to systemic hypertension. J. Appl. Physiol. 109 , 1996–1998 (2010).
Mark, A. L. The sympathetic nervous system in hypertension: a potential long-term regulator of arterial pressure. J. Hypertens. Suppl. 14 , S159–S165 (1996).
DiBona, G. F. Physiology in perspective: the wisdom of the body. Neural control of the kidney. Am. J. Physiol. Regul. Integr. Comp. Physiol. 289 , R633–R641 (2005).
Rafiq, K. et al . Renal sympathetic denervation suppresses de novo podocyte injury and albuminuria in rats with aortic regurgitation. Circulation 125 , 1402–1413 (2012).
Calaresu, F. R. & Ciriello, J. Renal afferent nerves affect discharge rate of medullary and hypothalamic single units in the cat. J. Auton. Nerv. Syst. 3 , 311–320 (1981).
Ciriello, J. & Calaresu, F. R. Hypothalamic projections of renal afferent nerves in the cat. Can. J. Physiol. Pharmacol. 58 , 574–576 (1980).
Katholi, R. E., Rocha-Singh, K. J., Goswami, N. J. & Sobotka, P. A. Renal nerves in the maintenance of hypertension: a potential therapeutic target. Curr. Hypertens. Rep. 12 , 196–204 (2010).
Ye, S., Ozgur, B. & Campese, V. M. Renal afferent impulses, the posterior hypothalamus, and hypertension in rats with chronic renal failure. Kidney Int. 51 , 722–727 (1997).
Converse, R. L. Jr et al . Sympathetic overactivity in patients with chronic renal failure. N. Engl. J. Med. 327 , 1912–1918 (1992).
Ecder, T. & Schrier, R. W. Hypertension in autosomal-dominant polycystic kidney disease: early occurrence and unique aspects. J. Am. Soc. Nephrol. 12 , 194–200 (2001).
Gabow, P. A. et al . Renal structure and hypertension in autosomal dominant polycystic kidney disease. Kidney Int. 38 , 1177–1180 (1990).
Klein, I. H., Ligtenberg, G., Oey, P. L., Koomans, H. A. & Blankestijn, P. J. Sympathetic activity is increased in polycystic kidney disease and is associated with hypertension. J. Am. Soc. Nephrol. 12 , 2427–2433 (2001).
Esler, M. D. et al . Renal sympathetic denervation in patients with treatment-resistant hypertension (The Symplicity HTN-2 Trial): a randomised controlled trial. Lancet 376 , 1903–1909 (2010).
Witkowski, A. et al . Effects of renal sympathetic denervation on blood pressure, sleep apnea course, and glycemic control in patients with resistant hypertension and sleep apnea. Hypertension 58 , 559–565 (2011).
Simões E Silva, A. C. & Flynn, J. T. The renin-angiotensin-aldosterone system in 2011: role in hypertension and chronic kidney disease. Pediatr. Nephrol. http://dx.doi.org/10.1007/s00467-011-2002-y .
Bhave, G. & Neilson, E. G. Body fluid dynamics: back to the future. J. Am. Soc. Nephrol. 22 , 2166–2181 (2011).
Graham, P. C. & Lindop, G. B. The anatomy of the renin-secreting cell in adult polycystic kidney disease. Kidney Int. 33 , 1084–1090 (1988).
Torres, V. E. et al . Synthesis of renin by tubulocystic epithelium in autosomal-dominant polycystic kidney disease. Kidney Int. 42 , 364–373 (1992).
Wang, E., Yayama, K., Takano, M. & Okamoto, H. Sexual dimorphism of urine angiotensinogen excretion in the rat. Jpn J. Pharmacol. 64 , 243–250 (1994).
Ingelfinger, J. R., Pratt, R. E., Ellison, K. & Dzau, V. J. Sodium regulation of angiotensinogen mRNA expression in rat kidney cortex and medulla. J. Clin. Invest. 78 , 1311–1315 (1986).
Lalouel, J. M. & Rohrwasser, A. Genetic susceptibility to essential hypertension: insight from angiotensinogen. Hypertension 49 , 597–603 (2007).
Thethi, T., Kamiyama, M. & Kobori, H. The link between the renin-angiotensin-aldosterone system and renal injury in obesity and the metabolic syndrome. Curr. Hypertens. Rep. 14 , 160–169 (2012).
Kang, J. J. et al . The collecting duct is the major source of prorenin in diabetes. Hypertension 51 , 1597–1604 (2008).
Xue, C. & Siragy, H. M. Local renal aldosterone system and its regulation by salt, diabetes, and angiotensin II type 1 receptor. Hypertension 46 , 584–590 (2005).
Kobori, H., Harrison-Bernard, L. M. & Navar, L. G. Expression of angiotensinogen mRNA and protein in angiotensin II-dependent hypertension. J. Am. Soc. Nephrol. 12 , 431–439 (2001).
Kobori, H., Nishiyama, A., Harrison-Bernard, L. M. & Navar, L. G. Urinary angiotensinogen as an indicator of intrarenal angiotensin status in hypertension. Hypertension 41 , 42–49 (2003).
Jang, H. R. et al . The origin and the clinical significance of urinary angiotensinogen in proteinuric IgA nephropathy patients. Ann. Med. 44 , 448–457 (2012).
Prieto-Carrasquero, M. C. et al . Enhancement of collecting duct renin in angiotensin II-dependent hypertensive rats. Hypertension 44 , 223–229 (2004).
Gilbert, R. E. et al . Pathological expression of renin and angiotensin II in the renal tubule after subtotal nephrectomy. Implications for the pathogenesis of tubulointerstitial fibrosis. Am. J. Pathol. 155 , 429–440 (1999).
Huang, L., Howard, C. G. & Mitchell, K. D. Chronic direct renin inhibition with aliskiren prevents the development of hypertension in Cyp1a1-Ren2 transgenic rats with inducible ANG II-dependent hypertension. Am. J. Med. Sci. http://dx.doi.org/10.1097/MAJ.0b013e3182410d1e .
Howard, C. G. & Mitchell, K. D. Renal functional responses to selective intrarenal renin inhibition in Cyp1a1-Ren2 transgenic rats with ANG II-dependent malignant hypertension. Am. J. Physiol. Renal Physiol. 302 , F52–F59 (2012).
Navar, L. G., Prieto, M. C., Satou, R. & Kobori, H. Intrarenal angiotensin II and its contribution to the genesis of chronic hypertension. Curr. Opin. Pharmacol. 11 , 180–186 (2011).
Kobori, H., Nangaku, M., Navar, L. G. & Nishiyama, A. The intrarenal renin-angiotensin system: from physiology to the pathobiology of hypertension and kidney disease. Pharmacol. Rev. 59 , 251–287 (2007).
Rautureau, Y. & Schiffrin, E. L. Endothelin in hypertension: an update. Curr. Opin. Nephrol. Hypertens. 21 , 128–136 (2012).
Clavell, A. L., Stingo, A. J., Margulies, K. B., Brandt, R. R. & Burnett, J. C. Jr. Role of endothelin receptor subtypes in the in vivo regulation of renal function. Am. J. Physiol. 268 , F455–F460 (1995).
Verhaar, M. C. et al . Endothelin-A receptor antagonist-mediated vasodilatation is attenuated by inhibition of nitric oxide synthesis and by endothelin-B receptor blockade. Circulation 97 , 752–756 (1998).
Kelland, N. F. et al . Endothelial cell-specific ETB receptor knockout: autoradiographic and histological characterisation and crucial role in the clearance of endothelin-1. Can. J. Physiol. Pharmacol. 88 , 644–651 (2010).
Ahn, D. et al . Collecting duct-specific knockout of endothelin-1 causes hypertension and sodium retention. J. Clin. Invest. 114 , 504–511 (2004).
Ge, Y. et al . Collecting duct-specific knockout of the endothelin B receptor causes hypertension and sodium retention. Am. J. Physiol. Renal Physiol. 291 , F1274–F1280 (2006).
Ge, Y., Stricklett, P. K., Hughes, A. K., Yanagisawa, M. & Kohan, D. E. Collecting duct-specific knockout of the endothelin A receptor alters renal vasopressin responsiveness, but not sodium excretion or blood pressure. Am. J. Physiol. Renal Physiol. 289 , F692–F698 (2005).
Koyama, H. et al . Plasma endothelin levels in patients with uraemia. Lancet 1 , 991–992 (1989).
Lehrke, I., Waldherr, R., Ritz, E. & Wagner, J. Renal endothelin-1 and endothelin receptor type B expression in glomerular diseases with proteinuria. J. Am. Soc. Nephrol. 12 , 2321–2329 (2001).
Goddard, J. et al . Endothelin A receptor antagonism and angiotensin-converting enzyme inhibition are synergistic via an endothelin B receptor-mediated and nitric oxide-dependent mechanism. J. Am. Soc. Nephrol. 15 , 2601–2610 (2004).
Goddard, J. et al . Endothelin-A receptor antagonism reduces blood pressure and increases renal blood flow in hypertensive patients with chronic renal failure: a comparison of selective and combined endothelin receptor blockade. Circulation 109 , 1186–1193 (2004).
Harris, R. C. et al . Cyclooxygenase-2 is associated with the macula densa of rat kidney and increases with salt restriction. J. Clin. Invest. 94 , 2504–2510 (1994).
Kömhoff, M., Grone, H. J., Klein, T., Seyberth, H. W. & Nüsing, R. M. Localization of cyclooxygenase-1 and -2 in adult and fetal human kidney: implication for renal function. Am. J. Physiol. 272 , F460–F468 (1997).
PubMed Google Scholar
Nantel, F. et al . Immunolocalization of cyclooxygenase-2 in the macula densa of human elderly. FEBS Lett. 457 , 475–477 (1999).
Brater, D. C., Harris, C., Redfern, J. S. & Gertz, B. J. Renal effects of COX-2-selective inhibitors. Am. J. Nephrol. 21 , 1–15 (2001).
Harding, P. et al . Cyclooxygenase-2 mediates increased renal renin content induced by low-sodium diet. Hypertension 29 , 297–302 (1997).
Yang, T. et al . Regulation of cyclooxygenase expression in the kidney by dietary salt intake. Am. J. Physiol. 274 , F481–F489 (1998).
Johnson, A. G., Nguyen, T. V. & Day, R. O. Do nonsteroidal anti-inflammatory drugs affect blood pressure? A meta-analysis. Ann. Intern. Med. 121 , 289–300 (1994).
Pope, J. E., Anderson, J. J. & Felson, D. T. A meta-analysis of the effects of nonsteroidal anti-inflammatory drugs on blood pressure. Arch. Intern. Med. 153 , 477–484 (1993).
Harris, R. C. COX-2 and the kidney. J. Cardiovasc. Pharmacol. 47 (Suppl. 1), S37–S42 (2006).
Whelton, A. Renal and related cardiovascular effects of conventional and COX-2-specific NSAIDs and non-NSAID analgesics. Am. J. Ther. 7 , 63–74 (2000).
Download references
Author information
Authors and affiliations.
Department of Transplantation, Mayo Clinic Florida, 4500 San Pablo Road, Jacksonville, 32224, FL, USA
Hani M. Wadei
Division of Nephrology and Hypertension, Department of Medicine, Mayo Clinic Rochester, 200 First Street SW, Rochester, 55905, MN, USA
Stephen C. Textor
You can also search for this author in PubMed Google Scholar
Contributions
Both authors contributed equally to discussion of content for the article, researching data to include in the manuscript, and reviewing and editing of the manuscript before submission. H. M. Wadei wrote the manuscript.
Corresponding author
Correspondence to Hani M. Wadei .
Ethics declarations
Competing interests.
The authors declare no competing financial interests.
Rights and permissions
Reprints and permissions
About this article
Cite this article.
Wadei, H., Textor, S. The role of the kidney in regulating arterial blood pressure. Nat Rev Nephrol 8 , 602–609 (2012). https://doi.org/10.1038/nrneph.2012.191
Download citation
Published : 28 August 2012
Issue Date : October 2012
DOI : https://doi.org/10.1038/nrneph.2012.191
Share this article
Anyone you share the following link with will be able to read this content:
Sorry, a shareable link is not currently available for this article.
Provided by the Springer Nature SharedIt content-sharing initiative
This article is cited by
Estimated glomerular filtration rate in observational and interventional studies in chronic kidney disease.
- Michele Provenzano
- Olga Baraldi
Journal of Nephrology (2024)
Contributions of renal water loss and skin water conservation to blood pressure elevation in spontaneously hypertensive rats
- Takahiro Ogura
- Kento Kitada
- Akira Nishiyama
Hypertension Research (2023)
Association between orthostatic blood pressure dysregulation and geriatric syndromes: a cross-sectional study
- Frédéric Roca
- Kevin Rougette
- Philippe Chassagne
BMC Geriatrics (2022)
The role of nitric oxide in renovascular hypertension: from the pathophysiology to the treatment
- Bruna Pinheiro Pereira
- Gabriel Tavares do Vale
- Carla Speroni Ceron
Naunyn-Schmiedeberg's Archives of Pharmacology (2022)
Association between pulse pressure, systolic blood pressure and the risk of rapid decline of kidney function among general population without hypertension: results from the China health and retirement longitudinal study (CHARLS)
- Huai-yu Wang
- Qinqin Meng
- Luxia Zhang
Journal of Translational Medicine (2021)
Quick links
- Explore articles by subject
- Guide to authors
- Editorial policies
Sign up for the Nature Briefing newsletter — what matters in science, free to your inbox daily.

Hot Topics in Kidney Health
Join us as we highlight the latest in kidney research, dispel myths, bring you up-to-date news in kidney care, and answer questions from patients to help them live well with kidney disease or a transplant.
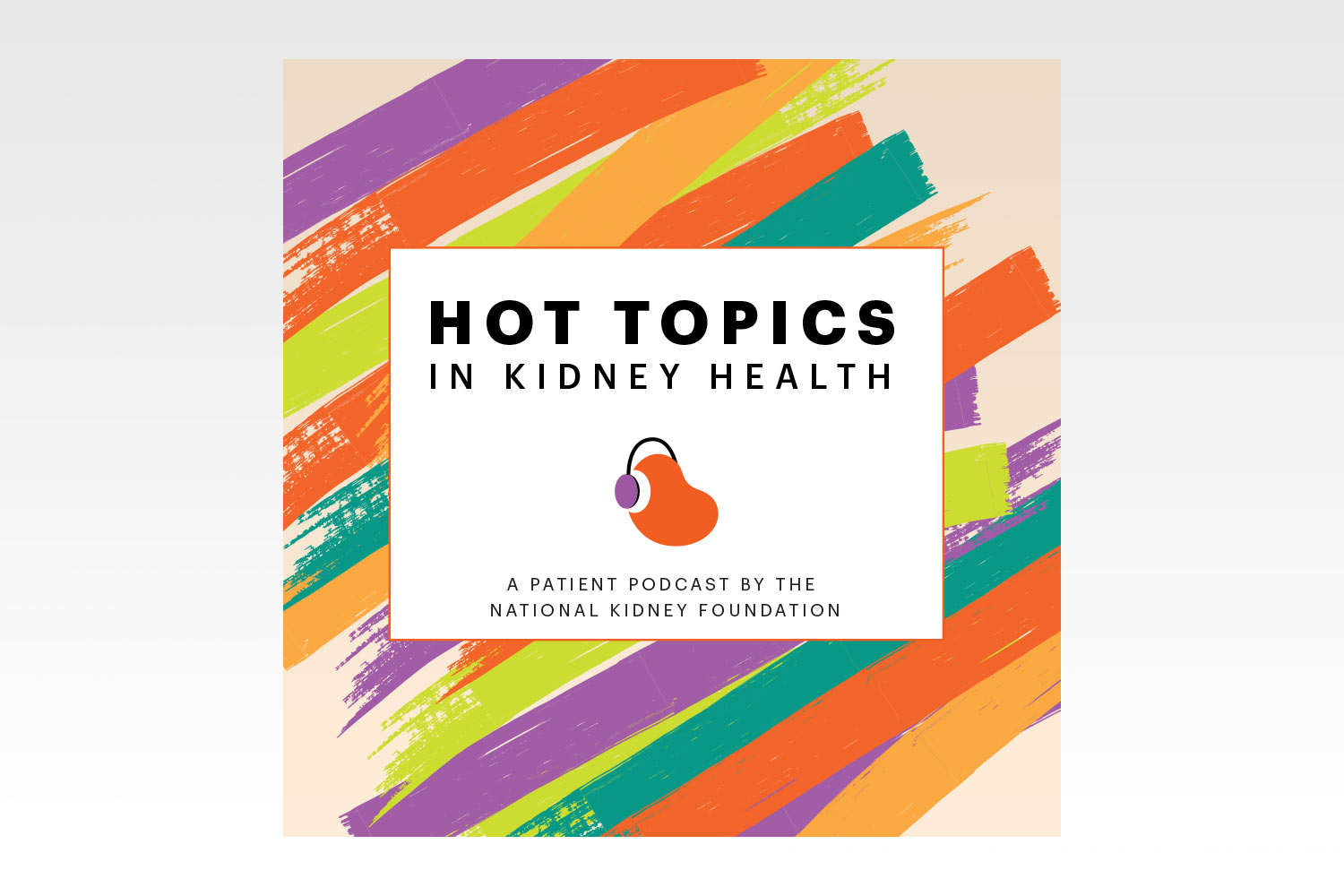
Subscribe on Apple Podcasts
Subscribe on spotify.
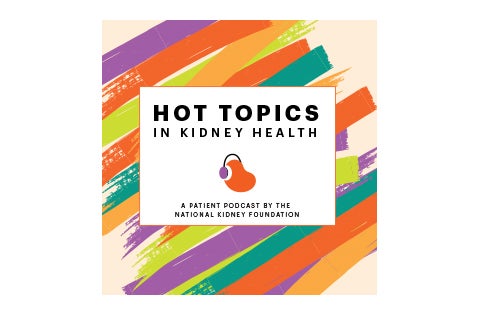
Power lifechanging research, education and advocacy to ensure that every person facing kidney disease gets the chance to thrive—not simply survive. Give today and help us provide FREE resources, support, and hope for patients, loved ones and healthcare heroes.
Unfiltered Story: Next steps after losing a transplant
Losing a kidney transplant can feel very overwhelming. Today we're here with Anthony Tuggle, a former NKF board member, to hear how he's coping with losing a transplant and learn what's next for him.
Coping with losing a transplant
Everyone loves to talk about kidney transplant success stories but rarely do we talk about what happens if a transplant fails. On today's episode Dori Muench, a post transplant social worker, and Sue George, a kidney warrior with experience losing a transplant, are here to discuss the impact of losing a kidney and how to cope.
Artificial Kidneys
When will the artificial kidney be ready for human use? Learn more about the progress of the artificial kidney and how it will affect someone's quality of life from expert Shuvo Roy, Ph.D.
What is a high KDPI kidney?
When a kidney from a deceased donor becomes available, it is scored on a system called the Kidney Donor Profile Index (KDPI). How accurate is it at predicting possible transplant survival? On this episode, we speak with experts to get the facts
Fad diets and kidney diets
It’s hard to keep up with all the new fad diets out there. Do you know which ones are right for you and your kidney health? On this episode, you will hear from experts on finding the diet that's right for you and how to stick to it.
Superfoods for people with kidney disease
You may have heard the buzzword superfoods but what are they and do they have special qualities? Board-certified renal dietitian, Jen Hernadez is here to break it down.
What do clinical end points and trials mean for CKD research?
Clinical trials exist to help prevent, screen for, diagnose, or treat diseases and other health problems. Without them, we would not have new treatments or other advances in health and medicine. But how are the clinical trial endpoints, or the preferred outcomes of these trials determined? Today, Anthony Gucciardo NKF’s Senior Vice President of Strategic Partnerships, Dr. Joseph Vassalotti, NKF’s Chief Medical Officer, and Kent Bressler, a Patient advocate and FSGS patient, discuss this and more.
Mindfulness meditation for kidney patients
You may have heard the term mindfulness before but what does it mean, what are the benefits, and how can you integrate mindfulness into your life? On today’s episode Gary Petingola a Social Worker certified to teach Mindfulness-Based Stress Reductionexplains all this and more.
Transplants for All
At the National Kidney Foundation, we believe that everyone who needs a kidney should get a kidney. To make this dream a reality, we’ve launched the Transplants for All Initiative. What is this initiative and how will it make a difference in the lives of people with kidney disease? In today’s episode special guests Morgan Reid, NKF’s Transplant Policy & Strategy Director and Haley Jenson, NKF’s Transplant Programs Director explain this and more.
BONUS: Sexuality and kidney disease
Sexual dysfunction is incredibly common in patients with kidney disease. In today's bonus episode, you'll learn about why it is so prevalent, the different treatment options, and how to improve communication around this important but often neglected aspect of health.
LGBTQ+ advocacy in healthcare
Over 50% of LGBTQIA-plus people have experienced some form of healthcare discrimination and over 25% of transgender people reported being denied care due to their transgender status. Not having proper health care or avoiding health care due to discrimination, can result in dire consequences, including an increased risk of health problems like kidney disease. What is this discrimination, and how can you advocate for yourself and LGBTQIA+ rights? In today’s episode Dr. Joshua Wilder, a podiatrist, and Representative Jeff Currey, two kidney transplant recipients and members of the LGBTQIA+ community, discuss this and more.
NKF Innovation Fund: developing new treatments for kidney patients
For far too long treatments for CKD haven’t changed. Launched in 2021, the NKF Innovation Fund works to accelerate funding, and development of therapies that kidney patients deserve. On this episode, you will hear from NKF CEO Kevin Longino interviews Kathleen St. Jean, Chief Commercial Officer of 34 Lives, one of the first recipients of a significant investment from NKF’s Innovation Fund to help further develop a new technology to rehabilitate donated kidneys for transplantation.
Living donors and mental health
Donating a kidney is one of the most selfless gifts one person can give to another. But what is that experience like for the donor before and after the transplant surgery? Today, we'll hear from Jessica Kolansky, a living kidney donor, and Alexandra Catalyst, a transplant coordinator, about the post transplant experience and what resources are available to donors after the surgery.
Laughter therapy for kidney patients
Is laughter really the best medicine? On today’s episode we’ll discuss an exciting new coping strategy for dialysis patients called guided group laughing therapy. What is it and how does it help? Dr. Paul Bennet who ran a study on the benefits of laughing therapy and Kimberly Super-Harrigan, a dialysis patient, are here to break it down.
BMI and weight management for kidney patients and living donors
Obesity is one of the major risk factors for developing kidney disease, which is measured through the body mass index or BMI. This measurement factors into the process for both living donors and transplant recipients. On today’s episode, you’ll learn how to measure your BMI and hear from both kidney patients on how managing their weight affected their kidney health.
Access to reproductive care for kidney patients
In 2022, the US Supreme Court overturned precedent established by Roe v Wade, eliminating the constitutional right to abortion. Family planning decisions can be difficult and complex for kidney patients. On today’s episode, we’ll discuss how this impacts kidney patients and disadvantaged patients with limited access to healthcare.
Game Changers: Treating CKD earlier
Did you know that 40% of chronic kidney disease progression may be preventable with earlier diagnosis and treatment? On this episode, you will hear the facts around treating CKD earlier from Dr. Joe Vassalotti, NKF’s Chief Medical Officer. You will also hear from Jane DeMeis on her journey with kidney disease.
Exercise and bone health: what CKD patients should know
Keeping your body and bones strong are important when you have kidney disease. Do you know what types of exercise are good for kidney patients? What about for transplant recipients or people on dialysis? In this episode, we sat down with experts to discuss the benefits and recommendations of managing an exercise routine.
Managing fluid intake as a CKD patient
It is important that certain individuals with CKD limit their fluid intake, but many don’t understand why or where to begin. On today’s episode, we sat down with experts to discuss fluid restriction, such as why it’s important, the negative effects of consuming too much, and ways to manage a fluid-restricted diet.
Treatment options for undocumented people with kidney disease
Undocumented people face increased barriers to accessing health coverage and care, including treatment for kidney disease. In this episode, our guest experts discuss what treatment options are currently available for undocumented people and what kind of advocacy efforts are being made to improve their access to health care.
Breaking Down New Medicare Coverage for Immunosuppressant Drugs
The Medicare coverage for immunosuppressive drugs for kidney transplant patients has recently been expanded. But what does this new benefit cover and who is eligible? in this podcast, Cynthia Nichols-Jackson, a registered nurse and a program coordinator for the National Kidney Foundation, and Troy Zimmerman, a special projects director for the National Kidney Foundation, discuss how this new policy will affect the future for kidney transplants.
Should You Follow A Special Diet After Transplant?
How potassium affects kidney patients
One of the jobs your kidneys is to manage your body’s levels of potassium, which keeps your heartbeat regular and your muscles working right. Many kidney patients need to watch what they eat to ensure that their potassium levels don’t become too high and cause dangerous health risks. On today’s episode, you’ll hear how to best manage your potassium levels to protect yourself.
How kidney patients should protect themselves from COVID-19 in 2022
While many people feel like the world is headed back to normal, many kidney patients can feel left behind. COVID-19 is still top of mind for those who are immunocompromised and we're here to offer resources and support. On today's episode, our guests will answer your questions about how COVID-19 affects transplant, dialysis, and early stage CKD patients.
What is basic science research and why is it important?
When we hear the word research, most of us think of innovative breakthrough treatments and technologies. But applied clinical research could not be possible without another type of research called basic science research in which scientists study the fundamental processes of the human body. In this episode, we’ll learn what basic science research is and how it applies to the area of kidneys and kidney disease.
Working with your clinician to make shared decisions for your care
Shared decision making can play a vital role in the treatment of kidney disease. When physicians involve patients in their care, they may be able to help slow progression and improve outcomes. In this episode, we discussed how patients and their physicians can achieve this and hear stories from patients who have been there.
How kidney disease affects your mental health
People with chronic conditions like kidney disease face mental health struggles such as depression or anxiety. In many kidney patients those challenges often go overlooked or undertreated. In this episode, we sat down with a mentor and mentee from NKF Peers to discuss their experiences and the importance of talking to someone who knows what you've been through.
Tips for finding a living donor
If you are in need of a kidney transplant, finding a living donor can sound scary and overwhelming. Where should you start and what’s the best way to share your story with the world? In this episode, you will hear from transplant recipients who once stood in your shoes.
Pig kidneys in humans? Xenotransplantation explained.
From the invention of the dialysis machine to the first successful kidney transplant, science has come a long way in extending life for kidney patients. Is transplantation between humans and animals the next step? In this episode, you will hear from a doctor behind the first successful transplant of a human receiving a pig kidney and where the science can take us from here.
Deciding to become a living donor
Pregnancy and kidney disease
Pregnancy can be an exciting time for most people, but what does it look like if you are a kidney patient? What does pregnancy look like if they have kidney disease, are on dialysis, or have received a transplant? In this episode, we’ll hear useful facts and tips from transplant nephrologist Dr. Mariana Markell, as well as personal stories from Cari Maxwell and Katie Reed, two mothers living with kidney disease.
What are SGLT2 Inhibitors and are they the right drug for you?
You might have heard about drugs called SGLT2 inhibitors used in treatment of kidney disease, but, just like many other kidney patients, you might not know if they’re right for you. On this episode, we explain how different SGLT2 inhibitors are from other kidney disease medications, discuss side effects, cost, and the ongoing research around this category of drugs.
How much sodium is safe for kidney patients?
Most patients on dialysis need to limit the amount of sodium in their diets. But how much sodium is safe and what are some ways to add flavor to your diet? On today‘s episode, our experts cover examples of high sodium foods, recommendations for low sodium substitutes, and how much sodium people with kidney disease should have as a part of a healthy diet.
What do changes to eGFR calculation mean for patients?
Tips for overcoming financial hardship for kidney patients
Many kidney disease patients often face financial hardships, such as having to leave a job or struggling to pay for medications. We sat down with physician and a postdoctoral research fellow Dr. Issac Acquah to talk about his recent research into the financial impact on people with chronic kidney disease.
How to become an advocate for kidney health
Over the past year, NKF advocates have won a number of policy campaigns both in Congress and state capitals across the country - but we're not done yet. In this episode, we discussed some recent wins and our upcoming challenges for the kidney advocate community with Jeff Currey, a Connecticut State Representative and kidney transplant recipient, and Armand Halter, an NKF patient advocate who helped lead NKF’s efforts to pass the Connecticut version of the Living Donor Protection Act.
A major worry for many people right now is the Delta variant, a highly contagious strain of COVID-19 which is making headlines across the United States. The FDA has just authorized a third dose of the COVID-19 vaccine so that immune compromised patients can better protecting themselves from the virus. What does this mean for CKD, dialysis, and transplant patients? We spoke with Dr. Joseph Vassalotti, NKF Chief Medical Officer, in a recent Facebook Live to share the facts about the Delta variant, COVID-19 booster shots, and other concerns facing kidney patients.
Coping with depression & anxiety during a global pandemic

A .gov website belongs to an official government organization in the United States.
A lock ( ) or https:// means you've safely connected to the .gov website. Share sensitive information only on official, secure websites.
- Risk Factors
- Caring for Yourself and Others
- Living with Chronic Kidney Disease
- Chronic Kidney Disease: Common, Serious, and Costly
- About the CKD Initiative
- Chronic Kidney Disease in the United States, 2023
- Chronic Kidney Disease Is Increasing in Mexican American Adults
- Publications
Chronic Kidney Disease Basics
- More than 1 in 7 American adults has chronic kidney disease (CKD).
- Specific blood and urine tests are needed to check for CKD.
- CKD can be treated (the earlier treatment starts the better).
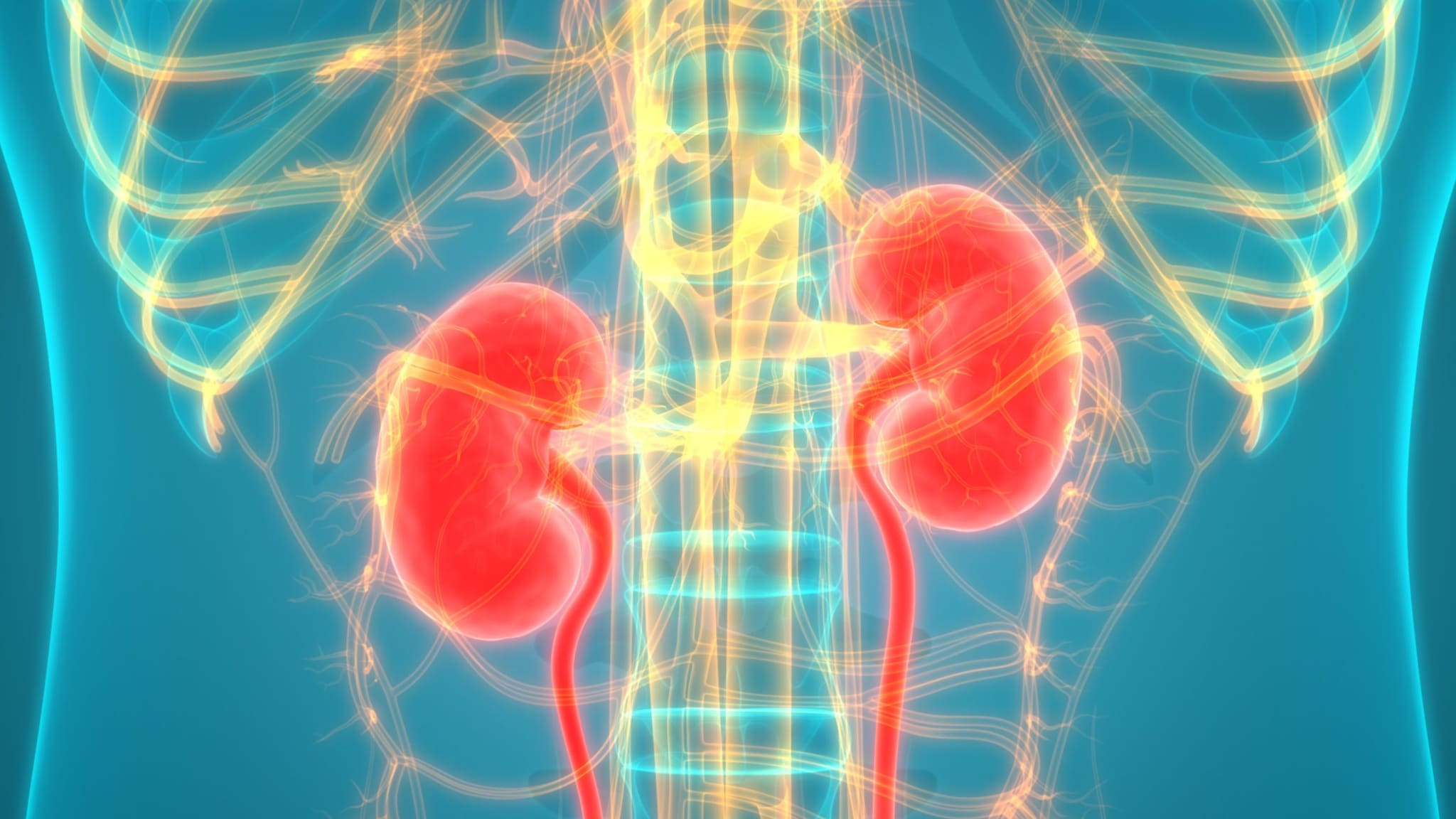
About your kidneys and CKD
Your hard-working kidneys.
Your kidneys, each just the size of a computer mouse, filter all the blood in your body every 30 minutes. They work hard to remove wastes, toxins, and excess fluid. They also:
- Help control blood pressure.
- Signal the body to make red blood cells.
- Help keep your bones healthy.
- Regulate blood chemicals that are essential to life.
Kidneys that function properly are critical for maintaining good health.
CKD is a condition in which the kidneys are damaged and can't filter blood as well as they should. Because of this, excess fluid and waste remain in the body and may cause health problems such as heart disease.
Other health problems related to CKD include:
- Anemia or low number of red blood cells.
- Increased occurrence of infections.
- Low calcium levels, high potassium levels, and high phosphorus levels in the blood.
- Loss of appetite or eating less.
- Depression or lower quality of life.
CKD has varying levels of seriousness. It usually gets worse over time, though treatment has been shown to slow progression. CKD can progress to kidney failure and early cardiovascular disease.
When the kidneys stop working, dialysis or kidney transplant is needed for survival. Kidney failure treated with dialysis or kidney transplant is called end-stage kidney disease. Not all people with kidney disease progress to kidney failure.
People with CKD may not feel ill or notice any symptoms. The only way to find out for sure if you have CKD is through blood and urine tests. These tests measure both the creatinine level in the blood and protein in the urine.
Reducing risk
- Keep your blood pressure below 140/90 mm Hg (or the target your doctor sets for you).
- If you have diabetes, stay in your target blood sugar range as much as possible.
- Get active. Physical activity helps control blood pressure and blood sugar levels.
- Lose weight if needed.
- Get tested for CKD regularly if you're at risk.
- If you have CKD, meet with a dietician to create a kidney-healthy eating plan. The plan may need to change as you get older or if your health status changes.
- Take medicines as instructed and ask your doctor about blood pressure medicines called angiotensin-converting enzyme inhibitors and angiotensin II receptor blockers, which may protect your kidneys in addition to lowering blood pressure.
- If you smoke, make a plan to quit . Smoking can worsen kidney disease and interfere with medication that lowers blood pressure.
- Include a kidney doctor (nephrologist) on your health care team.
CKD by the numbers
- Kidney diseases are a leading cause of death in the United States.
- About 35.5 million US adults are estimated to have CKD, and most are undiagnosed.
- 40% of people with severely reduced kidney function (not on dialysis) are not aware of having CKD.
- Every 24 hours, 360 people begin dialysis treatment for kidney failure.
- In the United States, diabetes and high blood pressure are the leading causes of kidney failure, accounting for 2 out of 3 new cases .
- In 2019, treating Medicare beneficiaries with CKD cost $87.2 billion , and treating people with end-stage kidney disease cost an additional $37.3 billion .
- Kidney Disease - Podcast (Running time 4 minutes 5 seconds)
- Native Americans, Diabetes, and Kidney Failure - Podcast (Running time 1 minute 14 seconds)
Get email updates
Chronic kidney disease.
The Chronic Kidney Disease (CKD) Initiative provides public health strategies for promoting kidney health.
For Everyone
Public health.
When kidneys get old: an essay on nephro-geriatrics
Affiliations.
- 1 Geffen School of Medicine, UCLA, USA.
- 2 Division of Nephrology and Hypertension, Mayo Clinic, USA.
- PMID: 28355403
- DOI: 10.5935/0101-2800.20170010
Aging is a nearly universal phenomenon in biology only partially controlled by genetic endowment. Individuals and their organs age at varying rates. The kidneys manifest the aging process by steady loss of nephrons and a corresponding decrease in glomerular filtration rate (GFR) beginning about age 30 years. The mechanisms responsible for this observation is are elusive. However, defining chronic kidney disease based on arbitrary, fixed thresholds of GFR in the later phases of life can be problematical as it may over-diagnosis CKD in the elderly. A modest, persisting reduction of GFR (around 45-59 ml/min/1.73m2) without abnormal proteinuria does not seem to confer much of an adverse effect on mortality and remaining life expectancy in older adults and the development of end-stage renal disease in such subjects is very uncommon. Old kidneys should not be equated with "diseased" kidneys.
Publication types
- Glomerular Filtration Rate
- Kidney / physiopathology*
- Kidney Failure, Chronic / etiology
- Open access
- Published: 28 July 2016
Interactions between kidney disease and diabetes: dangerous liaisons
- Roberto Pecoits-Filho 1 ,
- Hugo Abensur 2 ,
- Carolina C. R. Betônico 3 ,
- Alisson Diego Machado 2 ,
- Erika B. Parente 4 ,
- Márcia Queiroz 2 ,
- João Eduardo Nunes Salles 4 ,
- Silvia Titan 2 &
- Sergio Vencio 5
Diabetology & Metabolic Syndrome volume 8 , Article number: 50 ( 2016 ) Cite this article
97 Citations
15 Altmetric
Metrics details
Type 2 diabetes mellitus (DM) globally affects 18–20 % of adults over the age of 65 years. Diabetic kidney disease (DKD) is one of the most frequent and dangerous complications of DM2, affecting about one-third of the patients with DM2. In addition to the pancreas, adipocytes, liver, and intestines, the kidneys also play an important role in glycemic control, particularly due to renal contribution to gluconeogenesis and tubular reabsorption of glucose.
In this review article, based on a report of discussions from an interdisciplinary group of experts in the areas of endocrinology, diabetology and nephrology, we detail the relationship between diabetes and kidney disease, addressing the care in the diagnosis, the difficulties in achieving glycemic control and possible treatments that can be applied according to the different degrees of impairment.
Glucose homeostasis is extremely altered in patients with DKD, who are exposed to a high risk of both hyperglycemia and hypoglycemia. Both high and low glycemic levels are associated with increased morbidity and shortened survival in this group of patients. Factors that are associated with an increased risk of hypoglycemia in DKD patients include decreased renal gluconeogenesis, deranged metabolic pathways (including altered metabolism of medications) and decreased insulin clearance. On the other hand, decrease glucose filtration and excretion, and inflammation-induce insulin resistance are predisposing factors to hyperglycemic episodes.
Appropriate glycaemic monitoring and control tailored for diabetic patients is required to avoid hypoglycaemia and other glycaemic disarrays in patients with DM2 and kidney disease. Understanding the renal physiology and pathophysiology of DKD has become essential to all specialties treating diabetic patients. Disseminating this knowledge and detailing the evidence will be important to initiate breakthrough research and to encourage proper treatment of this group of patients.
The prevalence and incidence of diabetes mellitus (DM) has increased significantly worldwide, mainly due to a higher prevalence of type 2 DM. Type 2 DM globally affects 18–20 % of adults over the age of 65 years. It is estimated that approximately 285 million people, between 20 and 79 years old, currently have DM, 70 % of whom live in middle- and low-income countries. This increase in type 2 DM (DM2) occurs disproportionately, affecting mainly developing countries, thus bringing enormous challenges in the public health care for these patients. The expectation is for this number to increase by more than 50 % over the next 20 years if preventive programs are not implemented. By 2030, it is estimated that almost 438 million people, or 8 % of the adult population, will have DM [ 1 ].
Diabetic kidney disease (DKD) is one of the most frequent and dangerous complications of DM2, affecting about one-third of the patients. In addition to the increasing complexity of outpatient care for patients with DM, DKD results in increased hospitalizations and mortality rates, especially due to cardiovascular complications. DKD also increases the demand for renal replacement therapies, such as dialysis and kidney transplants. The combined economic and social costs of this disease are high and of concern to the world’s health systems.
Topics explored include pathophysiology, diagnostic measures, pharmacological and nonpharmacological treatments, and recommendations based on special considerations.
The discussion was divided into topics.
Pathophysiology of type 2 DM
DM2 is a disease characterized by persistent hyperglycemia, resulting from partial or complete insulin deficiency, and it is associated with a clinical picture of insulin resistance. Recently, other organs have been recognized as being involved in the pathogenesis of hyperglycemia in DM2, and it now known that not only dysfunction of the pancreas, but also of the liver, adipose tissue, intestine, kidneys, and central nervous system may contribute to this hyperglycemic state [ 2 ].
Insulin resistance (IR) is one of the pillars dictating the pathogenesis of DM2 and may differ according to body tissues. However, where does IR begin? Some authors argue it starts in the liver, others in the muscle, and others in the brain. What we know is that IR is present in various body tissues (liver, peripheral muscle, central nervous system, adipocytes, etc.) of patients with DM2, preventing glucose to entry into the cell and causing hyperglycemia. Several studies show that insulin has an anorexigenic action in the central nervous system [ 3 – 5 ]. However, the caloric intake in obese individuals is enhanced even in the presence of hyperinsulinemia, suggesting a clinical picture of IR in the brain [ 2 ]. Regarding peripheral IR, it is well established that IR directly correlates with deposits of visceral [ 6 ] and intramyocellular (within the myocyte) fat [ 7 , 8 ]. This can be explained by the inflammatory role of adipocytes in producing interleukin-6 and tumor necrosis factor-α, among other pro-inflammatory substances that alter intracellular signaling through the insulin receptor and consequently decrease the expression of glucose transporters of the cell membrane (GLUTs), leading to IR. In the muscle, when deposition of intramyocellular fat occurs, especially in the cytoplasm far from mitochondria, cytoplasmic diacylglycerol production increases, which leads to a decreased membrane expression of GLUT4, subsequent reduction of muscle glucose uptake, and hyperglycemia [ 7 ].
Hyperglycemia is not observed in a clinical picture of impaired glucose tolerance or pre-diabetes, since hyperinsulinemia can still compensate for IR and maintain normal levels of blood glucose. When hyperinsulinemia can no longer compensate for IR and insulin secretion begins to decline, the disruption of these variables results in hyperglycemia and a diagnosis of DM. In the early stages of DM2, the clinical picture of hyperinsulinemia persists. However, reduced insulin secretion is mainly responsible for the clinical picture of hyperglycemia. In the later stages of the disease, IR persists. However, the clinical picture, characterized by deficient insulin secretion, worsens, thus exacerbating the loss of glycemic control.
The gold standard to evaluate insulin resistance is the euglycemic insulin clamp technique. However, this technique is difficult to perform, expensive to apply, and is only used in clinical studies [ 9 ]. More simply, we can estimate IR using formulas that correlate with the clamp, such as the homeostatic model assessment (HOMA-IR): [fasting insulin (mU/mL) × fasting blood glucose (mmol/L)]/22.5 [ 10 ]. It is important to remember that HOMA-IR assesses hepatic IR, as the calculation involves the use of fasting blood glucose and insulin levels. On the other hand, the Matsuda index can estimate hepatic and peripheral insulin sensitivity, using glycemia and insulinemia values obtained through an oral glucose tolerance test [ 11 ].
In addition to IR, insulin deficiency is essential to manifest hyperglycemia in DM2. There are several factors involved in the process of insulin secretion, and incretins are one of the most important. Incretins are hormones secreted by the gut that have different functions upon binding to their receptors, expressed in various organs and tissues. After a meal, 60 % of insulin secretion depends on the stimulation of incretin hormones [ 12 ]. There are two main incretins: glucose-dependent insulinotropic peptide (GIP) and glucagon-like peptide-1 (GLP-1). Both are involved in glucose homeostasis. However, GLP-1 is more important than GIP, since it also inhibits glucagon secretion, slows gastric emptying, and inhibits hunger, whereas GIP does not [ 13 ]. Therefore, GLP-1 is a target of several incretin therapies for the treatment of DM2. GLP-1 is secreted by the L-cells of the ileum and has a half-life of 2 min; it is inactivated by dipeptidyl dipeptidase-4 enzyme [ 13 ]. When released into the circulation, GLP-1 binds to its receptor, which is expressed in different tissues, and promotes different actions. The GLP-1 receptor is a G-protein-coupled receptor, and binding activates adenylyl cyclase, leading to a subsequent increase in cyclic adenosine monophosphate, which activates protein kinase A and increases the release of insulin [ 14 ]. It is worth noting that incretins stimulate glucose-dependent insulin secretion, i.e., only if blood glucose is elevated. The GLP-1 receptor is expressed in multiple organs besides the pancreas, such as the intestine, kidneys, heart, and central nervous system. It exerts different functions in different tissues: (1) in the central nervous system, it decreases hunger and increases satiety; (2) in the pancreas, after a meal, it stimulates insulin secretion from β cells and decreases the release of glucagon by α cells; (3) in the liver, it reduces glycogenolysis and gluconeogenesis by decreasing postprandial glucagon; (4) in the heart, it plays a cardioprotective role; and (5) in the vessels, it acts as a vasodilator [ 15 ].
Patients with DM2 have an impaired incretin system, in addition to the dysfunction of β and α cells. A decreased effect of incretins affects not only the secretion of insulin, but also other beneficial effects promoted via the GLP-1 receptor. This supports several modern proposed DM2 pharmacological therapies aimed at improving the effects of incretins.
In addition to the pancreas, adipocytes, liver, and intestines, the kidneys also play important roles in glycemic control. In the tubular reabsorption of glucose, renal gluconeogenesis also contributes significantly to glucose homeostasis. By 1938, the first studies [ 16 ] on the role of the kidney in glycemic control were conducted in animals, and in the late 1950s, studies on renal glucose metabolism were conducted in humans [ 17 – 20 ]. Several hormones are involved in regulating renal reabsorption of glucose: hyperinsulinemia blocks the secretion of renal glucose as it does in the liver [ 21 , 22 ]. However, epinephrine infusion increases the release of renal glucose [ 23 ], and this effect is not altered by glucagon [ 24 ]. Although there is not yet available data in humans, several studies suggest that cortisol and growth hormone may stimulate the release of renal glucose [ 25 , 26 ].
Renal blood flow averages 1000–1500 mL/min, and in healthy individuals, all filtered glucose is reabsorbed by the renal tubules [ 27 ]. On average, the kidneys filter 162 g of glucose per day (considering a glomerular filtration rate of 180 L/day); 90 % of this is reabsorbed via the sodium/glucose cotransporter (SGLT) 2 expressed in the proximal tubule. The remaining 10 % of filtered glucose is absorbed by the SGLT1 transporter located in the descending proximal tubule, thus usually preventing glycosuria [ 2 ]. In individuals with DM, the Tm for glucose (maximum capacity of renal tubular reabsorption of glucose) is higher than in healthy individuals, thus worsening hyperglycemia. On average, the kidneys contribute 20 % of total body glucose through glucose tubular reabsorption and renal gluconeogenesis [ 27 ]. Because the kidneys play a role in glucose homeostasis, therapies have been developed to reduce tubular reabsorption of glucose, which is achieved by inhibition of the SGLT2 transporter. Other therapies suppress both SGLT2 and SGLT1, with the aim of improving glycemia by increasing glycosuria. Unlike previous views regarding glycosuria, after the introduction anti-SGLT1 and -SGLT2 medications in the treatment of DM2, glycosuria has become a desired clinical sign.
Glucose homeostasis in kidney disease
Glucose homeostasis is extremely altered in patients with CKD, who are exposed to a high risk of both hyperglycemia and hypoglycemia. Both high and low glycemic levels are associated with increased morbidity and shortened survival in this group of patients. Factors that are associated with an increased risk of hypoglycemia in CKD patients include decreased renal gluconeogenesis, deranged metabolic pathways (including altered metabolism of medications) and decreased insulin clearance. On the other hand, decrease glucose filtration and excretion, and inflammation-induce insulin resistance are predisposing factors to hyperglycemic episodes (Fig. 1 ). Appropriate glycaemic control tailored for diabetic patients is required to avoid haemodialysis-induced hypoglycaemia and other glycaemic disarrays [ 28 ].
Chronic kidney disease mechanisms predisposisng to hyperglycaemia and hypoglycaemia
Glycemic monitoring in CKD
As lack of glycemic control increases the rate of progression of renal failure, proper glycemic control in the early stages of CKD is crucial [ 29 ]. The United Kingdom Prospective Diabetes Study (UKPDS) provided the first evidence that intensive glycemic control, determined by a more aggressive therapy combined with monitoring and more frequent medical follow-up, could reduce the long-term complications caused by DM2 [ 30 ]. Although hyperglycemia is the biochemical marker of DM, hemoglobin A1c (HbA1c) has slowly become the cornerstone for the diagnosis and monitoring of DM since its introduction into routine clinical practice in 1976 [ 31 ]. There are confounding factors in the measurement of HbA1c, among which we have previously reported the difference in intracellular-extracellular glucose homeostasis, the survival time of red blood cells (hemolytic anemia), and non-glycemic genetic determinants of hemoglobin glycation. For this reason, the use of HbA1c as the only criterion for the diagnosis of DM in non-Caucasian individuals can lead to misclassification. In addition to its recent role as a diagnostic marker, HbA1c is also used to evaluate the degree of metabolic control in diabetics and to predict the risk of vascular complications [ 32 , 33 ].
There is conflicting evidence on the role of HbA1c in reflecting long-term glycemic control in patients with CKD. Moreover, the association between glycemic control and outcomes may be different in patients with or without CKD. Uremia causes a unique internal environment, which creates the need to assess each case in a personalized manner. Therefore, markers for monitoring glycemic control, specifically in individuals with CKD, need to be evaluated [ 34 ].
Glucose monitoring for the prevention of acute and chronic complications is critical in the management of DM. Therefore, we will discuss the main markers for glycemic control and their limitations in patients with CKD.
Blood glucose concentration
According to biological variation, in order to avoid patients misclassification, glucose measurement should have an analytical imprecision ≤2.9 %, a bias ≤2.2 %, and a total error ≤6.9 %. In a perfect scenario, glucose analysis should minimize total analytical error, and methods should be without measurable bias [ 35 ].
Enzymatic methods for glucose analysis are well standardized. A survey conducted by the College of American Pathologists (CAP) reveals that hexokinase or glucose oxidase is used in nearly all analyses performed in the U.S. few laboratories use glucose dehydrogenase. Glucose is stable for 8 h in samples collected with an antiglycolytic agent. In plasma, serum, and other liquids already separated from cells, the level of glucose is stable for 3 days at 2–8 °C if there is no bacterial contamination [ 36 ].
Important factors interfering with glucose measurement
Bilirubin levels >10 mg/dL produce negative interference when the endpoint method is utilized. For samples containing triglyceride concentrations >1100 mg/dL, the turbidity effect can be minimized using by diluting it with NaCl 150 mmol/L (0.85 %) and repeating the measurement. Bilirubin levels ≤10 mg/dL, hemoglobin ≤150 mg/dL, and triglycerides ≤3500 mg/dL do not produce significant interference when the kinetic method is used. Ascorbic acid at concentrations >100 mg/dL also interfere with the reaction, producing falsely low results [ 36 ].
Preparation for the exam
The collection should be preceded by a fasting period of 8–12 h, with water intake. Physical activity and a habitual diet are recommended the day before the examination as well as a standard diet of 150 g carbohydrates [ 36 ].
Home glucose monitoring or self-monitoring (SM)
SM is a valuable resource for both the patient and the doctor, as it is undoubtedly among the markers for glycemic control that provide the greatest amount of information about daily nutrition and the resultant glycemic responses. Pimazzoni et al. established several criteria that must be followed in order to produce favorable results, optimizing the use of SM [ 37 ]:
The patient should be instructed regarding the proper use and benefits provided by the correct SM practice.
The patient should follow a continued practice of DM education, not only in the initial discovery of the disease but also as the disease evolves.
There is no frequency of tests that can be recommended to all patients. On the contrary, this frequency should be individualized and adapted to the clinical conditions of each patient.
The SM results should be effectively used by the physician and other health professionals, to promote constant adjustments in the therapeutic conduct and supplementary guidance of nursing, nutrition, psychology, and physical education.
SM does not interfere with monitoring in diabetic patients with CKD. Its limitations include the need for training and economic access to tapes. However, there is no doubt that establishing a pattern of glycemic variation is fundamental. The importance of glycemic variability as an isolated factor for cardiovascular risk is well established [ 38 ]. Another important parameter regarding SM is the potential to download the information on specific software, generating accompanying graphs that facilitate understanding and decision-making.
Glycated hemoglobin
Glycation is a nonenzymatic reaction of glucose binding to a protein, in this case, to hemoglobin, yielding glycated hemoglobin, or HbA1c. In keeping with this notion, the term glycosylated hemoglobin is incorrect. The generic term “glycated hemoglobin” refers to a group of substances formed from the reactions between hemoglobin A (HbA) and certain sugars. This process is concentration- and time-dependent. In practical terms, this means that the greater the concentration of glucose available, the higher the concentration of HbA1c. In contrast, over time, there is a lower the binding of glucose to hemoglobin [ 39 ].
In contrast to plasma glucose, HbA1c represents nonenzymatic glycation, which depends on the concentration of glucose in the intra-erythrocytic compartment. Although several studies found a good positive correlation between the concentrations of HbA1c and glucose in diabetic patients with and without CKD, the variable relationship between HbA1c and estimated average glucose remains a potential source of concern [ 40 ].
It is interesting to notice that, normally, 97 % of hemoglobin is HbA. Only 6 % of HbA undergoes a glycation process and becomes HbA1c. Ninety-four percent of HbA undergoes no action induced by any sugars and is called HbA0. In turn, HbA1c is divided into subtypes in accordance with the type of sugar that produces glycation. Twenty percent of HbA1c is influenced by fructose-1,6-diphosphate and glucose-6-phosphate, forming HbA1a and HbA1b. The remaining 80 % of HbA1c is glycated dependent upon glycemic variation and is called HbA1c [ 39 ].
Main laboratory methods used
The following methods are approved by the National Glycohemoglobin Standardization Program (NGSP): high performance liquid chromatography (HPLC—a method that was applied in the diabetes control and complications trial (DCCT)), boronic acid affinity chromatography, enzymatic, immunoassay, and capillary electrophoresis. Since different methods quantify different ratios of glycated hemoglobin, the results are different. However, an excellent correlation was observed in a sample without hemoglobin variants or the presence of interfering factors. Through NGSP, the values of glycated hemoglobin can be expressed to provide equivalent results of the glycemic status of the patient, regardless of the method used, thus the same criteria can be widely applied. NGSP standardized these methods for the results to be comparable to those obtained by DCCT, in which the relationship between the average levels of blood glucose and the risk for vascular complications was established. A list of methods and worldwide laboratories, the certification of which depends on the demonstration of an acceptable accuracy and compliance with DCCT standards, is also provided on their website ( http://www.ngsp.org ) [ 41 ].
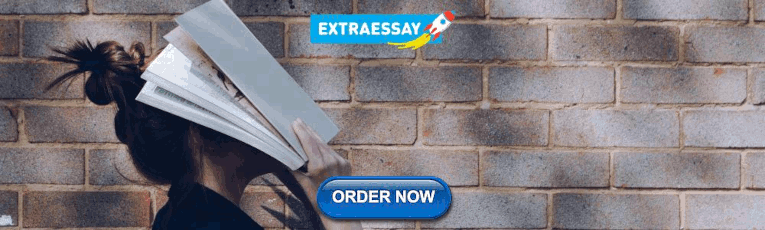
Important factors causing technical interference
Interference in the dosage of HbA1c might occur and depends on the method used: factors increasing HbA1c measurements include renal impairment (increased urea binds to hemoglobin, producing carbamylated hemoglobin that interferes with HbA1c measurement); use of acetylsalicylic acid (binds to hemoglobin, producing acetylated hemoglobin, which interferes with HbA1c measurement; usually, this occurs with high doses of acetylsalicylic acid); hypertriglyceridemia; and hyperbilirubinemia. Finally, factors decreasing HbA1c measurements include hemoglobin glycation inhibition factors (e.g., vitamins C and E) [ 42 ].
Clinical conditions that interfere with the method
Interference might occur with the dosage of HbA1c, depending on the method: factors increasing HbA1c measurements include polycythemia, anemia due to iron deficiency, folic acid, or vitamin B12; chronic alcoholism; and opiates. Factors decreasing HbA1c measurements include conditions that shorten the half-life of red blood cells (e.g., hemolytic anemia, hemorrhages), lead poisoning, erythropoietin deficiency secondary to renal failure, multiple myeloma, hyperthyroidism, leukemia, and severe burns with loss of fluid and proteins [ 42 ].
Fasting is not necessary for the collection of the material. Whole blood collected using EDTA as anticoagulant. The blood can be stored in a refrigerator for a week. Heparinized samples should be assayed in a maximum of 48 h [ 41 ].
Limitations of glycated hemoglobin in CKD
In addition to glucose, other factors might also influence HbA1c: this is the main reason for which the dosage of HbA1c is questioned in patients with CKD. Among these influences, we highlight a few. First, the formation of HbA1c is dependent on the interaction (intensity and duration) between the concentrations of glucose and blood erythrocytes. On average, erythrocytes survive 117 days in men and 106 days in women. At a certain point, a blood sample contains erythrocytes of different ages, mainly younger elements and with different degrees of exposure to hyperglycemia [ 40 ]. HbA1c is a measure for the mean level of blood glucose in the past 90 days. The impact of recent blood glucose levels on the measurement of A1c are: 50 % for the last month, 25 % for the 2nd month ago, and 25 % for the 3rd and 4th month ago.
An unexplained discrepancy between HbA1c and other measurements of glycemic control can be partly due to the different life span of erythrocytes. Decreased erythropoiesis, caused by iron or vitamin B12 deficiency or aplastic anemia, leads to an increased number of aged red blood cells and a subsequent progressive increase of HbA1c, unrelated to glycemic control [ 43 ].
Anemia due to iron deficiency increases HbA1c up to 2 %, which can be reverted by iron supplementation. Conversely, a decrease in HbA1c is observed after the administration of erythropoietin, iron, and vitamin B12, and in cases of hemolytic anemia. Due to a reduction in the survival of red blood cells, younger erythrocytes have less time to be exposed to a glycemic environment and therefore undergo less glycation [ 44 ] Hemoglobinopathies, of which the most common example is sickle cell anemia and thalassemia, can lead to problems in the interpretation of HbA1c. In case of these changes, in addition to the normal HbA0 glycation to form HbA1c, other glycation products derived from HbC (African populations), HbD (indigenous populations), HbE (Asian populations), or HbS (sickle cell anemia) could be formed [ 43 ].
In the third National Health and Nutrition Examination Survey, alcohol consumption was associated with low levels of HbA1c in 1024 adults with DM. These findings were confirmed in a large follow-up study of 38,564 adult patients with type 1 or 2 DM. An increase in alcohol consumption predicts lower HbA1c values. Also, the intra-erythrocytes pH can interfere HbA1c. In patients with chronic renal failure, lipid peroxidation of Hb can increase hemoglobin glycation. Chronic ingestion of aspirin and high doses of antioxidants (e.g., vitamins C and E) may reduce HbA1c, since they inhibit glycation. It is unclear whether these phenomena could change clinical practice [ 45 ].
In addition to the changes described above, it is important to highlight that new methods detect differently the presence of hemoglobinopathies, and the presence of carbamylated hemoglobin can interfere with the dosage. HbA1c measured by HPLC detects the carbamylated fraction differently than does immunoturbidimetry, which does not identify this fraction; consequently, patients with renal failure present higher levels of HbA1c when measured using HPLC [ 44 ].
Glycated albumin
The dosage of glycated albumin (GA) is gaining interest as a potential marker of glycemic control. GA is a ketoamine formed by the non-enzymatic oxidation of albumin by glucose. As the half-life of albumin is of approximately 15 days, GA is used as a short-term measurement of glycemic control, that is, 2–3 weeks, and as such, it might act as an intermediate time index of glycemic control [ 44 ].
Several methods can be used for the measurement of GA, including affinity chromatography, ion-exchange chromatography, HPLC, immunoassay techniques, capillary electrophoresis, and other electrophoretic and enzymatic assays. It is not influenced by sex, red blood cell life span, or erythropoietin therapy; however, for serum albumin concentration, the results are conflicting [ 46 ].
However, the results can be influenced by age, nutritional status, albuminuria, cirrhosis, thyroid dysfunction, and smoking. GA is inversely influenced by body mass index, body fat mass, and visceral adipose tissue [ 46 ].
Glycated fructosamine
Fructosamine is the generic name given to all glycated proteins, of which albumin is the major plasma fraction, after hemoglobin. Although the dosage of fructosamine can be automated, thus making it cheaper and faster than HbA1c measurement, there is no consensus on its clinical utility [ 47 ].
The level of fructosamine correlates better with the average glucose levels over the previous 10–14 days. As this is a measure of total glycated serum proteins, of which glycated albumin represents approximately 90 %, fructosamine concentrations can be influenced by the concentrations of serum proteins and the profile of different proteins [ 47 ].
Moreover, fructosamine is influenced by the concentration of bilirubin and substances with low molecular weight, such as urea and uric acid. GF is not modified by changes in the metabolism of hemoglobin. However, it is affected by disturbances in protein turnover. The reference values depend on age, sex, sample population, and test method applied [ 48 ].
Unfortunately, the data show conflicting results concerning the correlation between fructosamine and glucose concentrations in patients with CKD. The values may be influenced by nephrotic syndrome, thyroid diseases, administration of glucocorticoids, liver cirrhosis, and jaundice [ 48 ].
1,5-Anhydroglucitol (1.5-AG)
1.5-AG is another blood glucose marker and is a naturally occurring dietary plasma polyol, for which levels are maintained constant during normoglycemia by filtration and renal reabsorption. The physiological function and metabolism of 1,5-AG are not well defined. 1,5-AG is a non-metabolizable glucose analog found in plasma after food intake. It is characterized by urinary excretion, filtration through the glomeruli at a rate of 5–10 g/L, and high tubular reabsorption (>99 %), which is inhibited by glucose during periods of hyperglycemia [ 49 ].
The levels of 1,5-AG in blood are altered less than 24 h after hyperglycemic episodes, and the repetition of these episodes dramatically decreases its concentration. The values of 1,5-AG reflect hyperglycemia over a period of approximately 1 week. In addition to the glycosuria threshold, the measurement of 1,5-AG could play an adjuvant role in the control of DM, especially as a short-term single marker for hyperglycemia excursions [ 50 ].
The relationship between HbA1c and glucose is more complex in more advanced stages of CKD due to the great variability in hemoglobin, nutritional status, and inflammation. Moreover, these underlying comorbidities may also hinder the prognostic value of HbA1c [ 44 ].
Figure 2 shows the correlation between each marker and the time of hyperglycemia that each indicates.
Correlation between each marker and the time of hyperglycemia that each indicates
Current guidelines recommend using HbA1c as preferred biomarker for glycemic control in patients with CKD, with a goal of 7 % to prevent or delay the progression of microvascular complications of DM, including diabetic nephropathy [ 33 ]. However, these guidelines refer mainly to the initial stages of CKD. In diabetic patients with advanced disease, it is suggested that the objective of a very intensive glycemic control, HbA1c <6.5 %, can be associated with increased mortality.
A cohort study assessing 54,757 diabetics on hemodialysis demonstrated that an average HbA1c >8 % or an average glucose >200 mg/dL seemed to be associated with an increased cardiovascular mortality [ 51 ]. A recent meta-analysis, investigating the relationship between HbA1c and risk of death in diabetic hemodialysis patients, showed that the level of HbA1c remains a useful clinical tool for the prediction of the mortality risk [ 52 ].
Although glycated albumin presents advantages in patients with CKD, several authors argue that CKD is characterized by the disruption of albumin homeostasis and that the threshold of serum albumin for which the risk of death increases varies according to the dialysis modality [ 53 ]. In the presence of hypoalbuminemia, plasma protein glycation is increased. However, glycated albumin seems to reflect the percentage of albumin that is glycated, regardless of the concentration of total serum albumin, although more studies on a large scale with dialysis patients would be required to confirm this observation [ 54 ].
Glycated albumin seems to be a better marker to reflect the accuracy of glycemic control when compared to HbA1c in patients with DKD. However, due to limited data, the absence of studies on the results of interventions based on glycated albumin and its expensive and laborious methodology, indicate that it might be premature to abandon HbA1c in favor of glycated albumin [ 55 ].
Thus, our recommendation is that diabetic patients with CKD would be monitored in the best possible way, in the attempt to prevent the progression of the disease and an increase in complications. Therefore, we suggest monitoring HbA1c every 3 months, which can be associated with home SM when possible. Other exams such as glycated fructosamine, glycated albumin, and 1,5-AG could be used as additional tools, rather than replacing HbA1c.
General approach of DM treatment in CKD
General considerations for the control of dm in ckd.
Glycemic control is fundamental in the prevention and progression of complications associated with DM [ 56 , 57 ]. Studies show that reducing HbA1c to values ≤7 % influences the reduction of microvascular complications caused by DM, and if implemented early, it is also associated with a reduced occurrence of macrovascular complications [ 56 , 57 ].
The goals proposed by the Brazilian Diabetes Society (SBD) in 2013/2014 Guidelines recommend achievement of the following aims: fasting glucose <100 mg/dL, preprandial glycemia <130 mg/dL, postprandial glycemia ≤160 mg/dL [ 58 ], and HbA1c <7 %. In 2015, The American Diabetes Association (ADA) reinforced its proposal to keep HbA1c optimal values <7 % for most diabetic adults [ 59 ]. However, in recent years, the associations focused on the treatment of DM have systematically reviewed the optimal values of glycemia and HbA1c goals for diabetic patients, with the aim to define individualized objectives to prevent the onset of chronic complications, aiming also to reduce the occurrence of hypoglycemia.
The ACCORD (action to control cardiovascular risk in diabetes) trial was a landmark in demonstrating that patients with high cardiovascular risk, when treated intensively with the aim to achieve HbA1c of approximately 6 %, presented an increased risk of death [ 60 ]. After this study, associations such as ADA began to recommend individualized HbA1c goals for patients with a history of severe hypoglycemia, limited life expectancy, patients with microvascular or macrovascular complications in advanced stages, and patients with multiple comorbidities. The recommendation of less strict HbA1c goals (around 8 %) for these groups aims to reduce the morbidity and mortality associated with a very strict glycemic control, often related to an increase in hypoglycemic episodes [ 59 ].
Specifically in relation to DKD, classical studies have also previously demonstrated that improved glycemic control is associated with a reduced incidence of albuminuria in both type 1 and type 2 DM [ 56 , 57 ]. Even in secondary prevention, i.e., when the kidney disease is already established, glycemic control remains a major therapeutic weapon to combat the progression of CKD [ 61 , 62 ]. The ADVANCE (action in diabetes and vascular disease) trial showed that intensive control was able to reduce albuminuria, nephropathy, and the need for hemodialysis [ 63 ]. Similarly, the ACCORD trial showed a significant reduction in albuminuria (although not in advanced renal disease) in the group treated with an intensive therapy for glycemic control [ 60 ].
However, despite evidence correlating the optimization of glycemic control to the benefits observed in the evolution of DKD, glycemic and HbA1c objectives are very difficult to define and achieve in this population. The complexity of glycemic control in this group of patients is explained not only by the metabolic alterations associated with DKD, but also the specificity and greater difficulty in the use of hypoglycemic drugs, difficulty in monitoring glycemic levels, behavioral addictions related to years of DM and a fear of hypoglycemia, as well as sociocultural and economic factors.
DKD progresses with several metabolic changes, which occur concomitantly with the progressive decline in glomerular filtration rate (GFR). Using the euglycemic insulin clamp, DeFronzo et al. showed that the glucose used by peripheral tissues in response to insulin is reduced in uremia [ 64 ]. The increased insulin resistance is related to the accumulation of uremic toxins, markers of chronic inflammation, increased visceral fat, oxidative stress, and vitamin D deficiency. Progression to uremia is associated with decreased insulin sensitivity of peripheral tissues, increased hepatic gluconeogenesis, decreased glucose uptake by skeletal muscle cells, and deficiency of intracellular glycogen synthesis and subsequent hyperglycemia [ 65 ]. On the other hand, the risk of hypoglycemia is a constant concern, since this is increased in diabetic patients with CKD. The pathogenesis of hypoglycemia in these patients is related to changes in glucose metabolism, decreased insulin degradation, and changes in the metabolism of hypoglycemic agents. With a progressive reduction in GFR, we observed a decrease in the clearance of oral hypoglycemic agents, and sometimes, a longer time of action of these drugs and their active metabolites. Similarly, insulin metabolism is also altered, since part of its metabolization and excretion is carried out by the renal system [ 66 – 68 ]. A restricted diet, either by prescription or even due to uremia, reduces hepatic gluconeogenesis, thus contributing to the occurrence of hypoglycemic episodes observed at higher frequency in this population [ 69 , 70 ].
Therefore, since CKD is a condition that increases predisposition to hyperglycemic and hypoglycemic peaks, the choice of drug treatment for these patients should be carefully considered [ 71 – 73 ]. Most classes of oral hypoglycemic agents should be avoided when GFR is <40 mL/min, which indicated a higher risk of hypoglycemia. Insulin is the therapy of choice for the treatment of diabetic patients with advanced CKD, and for insulinization to occur properly. Adherence and understanding of patients are of utmost importance. In phases IV and V of CKD, almost all patients with DKD (in which DM is the central determinant in the etiology of DKD) need insulin. Patients with advanced CKD in which DM is another comorbidity, rather than the etiology of CKD, require insulin less frequently. Therefore, it is important that attending physicians have a broad knowledge of the arsenal of oral hypoglycemic agents that are currently available, in order to avoid the use of insulin when possible and the inappropriate and dangerous use of oral hypoglycemic agents. Oral hypoglycemic agents could also be used in patients with burnout syndrome, in which the “disappearance” of DM is almost always observed because of important homeostatic changes related to diet restrictions, catabolism, weight loss and greater circulation of endogenous insulin.
In any case, most patients with advanced CKD need to use insulin for the safe and effective control of DM. However, for this to be achieved, a number of points should be discussed with the patient and the family:
Proper storage of insulin
Application techniques, insulin mixing techniques, and rotation of daily application locations
Strict diet at pre-determined times
Guidelines on how to proceed in the presence of hypoglycemia
Adherence to multiple daily insulin injections
Conduction of pre- and postprandial capillary blood glucose tests, also conducted at dawn, facilitating dose adjustment.
These guidelines require a commitment not only from the patients and their families, but also from a multidisciplinary team to make certain that the procedures are fulfilled. It is known that many diabetic patients who evolve towards a progressive loss of renal function have a personal history of poor adherence to the treatment, either due to inherent factors of the patient or the difficulty of the health system in dealing with a complex framework, thus demanding specific care. We also noticed that many patients with advanced stage kidney disease often have comorbidities that further hamper their adherence to the treatment. Patients with diabetic retinopathy (DR) or those who have undergone amputation require the support of their families for periodic consultations, drug administration, and completion of capillary blood glucose monitoring tests.
The awareness and motivation of the patients and their families to complete the proposed treatment strategies in order to achieve the necessary goals for proper metabolic control should always be reviewed and emphasized by the multidisciplinary team. It is important that the entire team pays attention in identifying the problems that can range from understanding the subject, to access to information and inadequate use of insulin. These habits are particularly common in patients with a history of poor glycemic control caused by self-medication for many years or by extreme fear of hypoglycemic episodes that led to the use of lower doses of insulin (most often not disclosed to the medical team). A condition often observed in populations of lower socioeconomic conditions is concurrent very high glycated hemoglobin levels and frequent episodes of hypoglycemia. Therefore, the best option is to provide DM re-education, review dietary patterns, and ensure fractionation of insulin dosage. Often, however, the medical team responds inadequately, and insists on increasing the insulin dose, which the patient reduces without reporting the decrease because of fear of worsening hypoglycemia. This creates a complete dissociation between the healthcare team and patient, with mutual loss of trust and overall inefficacy of the treatment. If this occurs, the process of re-education becomes even more important, since in addition to directly approaching patients and their families, it becomes necessary to work on concepts, insecurities, and prescription patterns of the attending medical team.
According to NKF–KDOQI (National Kidney Foundation–Kidney Disease Outcomes Quality Initiative), HbA1c objectives in diabetic patients with CKD do not differ from those recommended for patients without renal disease, aimed to maintain HbA1c values lower than 7 % [ 74 – 76 ]. However, as already mentioned, the importance of individualization of HbA1c goals has already recognized by the ADA [ 59 ]. It is noteworthy that most diabetic patients with CKD or DKD, in a broad sense, fit the ADA’s criteria for high risk of hypoglycemia.
Hypertension, dyslipidemia, and other microvascular complications in diabetic patients with CKD
Blood pressure control is fundamental in the management of kidney disease progression. In general, diabetic patients with lower blood pressure levels and renal disease tend to experience slower progression of the pathology compared to hypertensive patients with the same condition [ 77 ]. Non-pharmacological measures (dietary changes and increased physical activity) have an impact on blood pressure control and should be encouraged. Drugs inhibiting the renin-angiotensin system through its specific renoprotective effect, regardless of the reduction in systemic blood pressure, have a well-established role in diminishing albuminuria and DKD progression [ 78 ].
Studies comparing the effect of angiotensin-converting enzyme (ACE) inhibitors and angiotensin II receptor blockers (ARBs) reported similar effectiveness. Therefore, ACE inhibitors or ARBs are recommended in patients with CKD, regardless of their ethnicity, as first-line treatment or in combination with another antihypertensive drugs [ 79 ]. Dose adjustment for these agents should be gradual, with periodic assessment of renal function and potassium levels, since there is a risk for creatinine level elevation and hyperkalemia. Greater attention must be paid to monitoring elderly patients and individuals with advanced stage CKD. In December 2013, the 8th Joint National Committee of Hypertension discussed new strategies for blood pressure control, and it was recommendation that ACE inhibitors and ARBs should not be used in the same patient simultaneously due to the following concerning findings: first, the VA-NEPHRON D trial [ 80 ] was prematurely terminated because of concerns about a high prevalence of hypotension, hyperkalemia and acute kidney injury with dual renin-angiotensin system (RAS) therapy. Actually, these adverse events could have been prevented by avoiding forced ACEi up-titration in patients with an eGFR as low as 30 mL/min/1.73 m2 on top of full-dose ARB. Notably, at study closure dual versus single RAS inhibition had already reduced end-stage renal disease events by 34 %, a treatment effect never reported before in type 2 diabetes. Risk reduction was associated with a significantly greater decline in proteinuria and approached nominal significance (P = 0.07) over just 2.2 years of follow-up. Second, in the RENAAL study [ 78 ], performed in type 2 diabetic patients, the larger antiproteinuric effect of losartan was associated with a similar (28 %) end-stage renal disease reduction compared to placebo. The treatment effect was, however, not still appreciable at 2.2 years, but became statistically significant over the planned 3.2 years of follow up. These data strongly suggest that also in the VA NEPHRON-D trial, end-stage renal disease events could have been significantly reduced over the initially scheduled 5-year study period. Consistently, the results of a recent meta-analysis showing that dual RAS blockade with ACE inhibition and ARB is the most effective strategy to prevent end-stage renal disease in patients with diabetes and kidney disease [ 81 ].
The development of objectives to achieve adequate blood pressure levels to reduce cardiovascular events and progression of kidney disease has been the goal of recent studies. The ACCORD trial failed to show a reduction in cardiovascular events; moreover, in the ACCORD study [ 77 ], there were significantly more instances of an eGFR less than 30 mL/min/1.73 m 2 in the intensive-therapy group than in the standard-therapy group (P < 0.001), although only 38 participants in the intensive-therapy group and 32 in the standard-therapy group had two or more instances of eGFR <30 mL/min/1.73 m 2 (P = 0.46). The frequency of macroalbuminuria at the final visit was significantly lower in the intensive-therapy group than in the standard-therapy group, and there was no between-group difference in the frequency of end-stage renal disease or the need for dialysis. In addition, the INVEST study also showed no mortality reduction in patients with a desired systolic blood pressure <130 mmHg compared to that in patients with systolic blood pressure 130–139 mmHg [ 82 ].
Optimal blood pressure values have not been established. However, in 2015, the ADA aligned its recommendations with hypertension guidelines, recommending the maintenance of a systolic blood pressure lower than 140 mmHg and diastolic pressure below 90 mmHg as goals for the treatment of hypertensive diabetic patients [ 59 ]. Similarly, the 8th Joint National Committee of Hypertension also recommends that blood pressure for diabetic patients and individuals with CKD should be <140/90 mmHg [ 79 ].
Additional positive phase 2 clinical studies with drugs that have hemodynamic actions such as endothelin antagonists and mineralocorticoid receptor antagonists have led to larger phase 3 trials with atrasentan and finerenone, respectively, in order to address if these drugs indeed delay the development of end-stage renal disease [ 83 ]. Positive findings with respect to new glucose-lowering agents such as sodium-dependent glucose transporter 2 inhibitors may lead to a change in the way we treat diabetic individuals with or at risk of DKD. A number of other pathways are currently under active preclinical investigation and hopefully over the next decade will lead to promising drug candidates for subsequent clinical trials [ 83 ].
DM and CKD present a significant correlation with increased cardiovascular risk. The risk of events in patients with CKD is considered equivalent to that in patients with a history of coronary disease. Therefore, the combination of these two conditions classifies the patient with DKD as presenting a very high risk for a cardiovascular event. Considering the exacerbated cardiovascular risk of these patients, kidney disease: improving global outcomes (KDIGO) does not recommend the use of routine low-density lipoprotein (LDL) cholesterol level testing to identify patients to be treated or the objectives of the treatment [ 84 ].
The current recommendation indicates the use of statins as drugs of choice since their efficacy in primary and secondary prevention of cardiovascular events has been proven, regardless of LDL levels [ 76 , 84 ]. However, the appropriate dosage remains controversial. While ADA recommends the use of statins in high doses for diabetic patients with risk factors for cardiovascular disease, KDIGO recommends the reduction of the dosage of statins in individuals with a GFR lower than 60/mL/min/1.73 m 2 [ 59 , 76 , 85 , 86 ]. This recommendation is based on the reduction of renal excretions (valid for some statins) and associated comorbidities. However, no studies have shown an increase in adverse events using high doses of statins, and the prescription information of atorvastatin states that there is no need for a dose adjustment in patients with CKD [ 85 ]. On the other hand, it is known that patients with CKD have an increased risk of muscle damage with the use of statins, therefore this group of patients should be monitored more carefully. Results of studies on the use of statins in individuals undergoing dialysis, in whom the cardiovascular risk is very high, have been disappointing. Despite the high risk, the cardioprotective effect of statins seems to be less efficient than in other populations. Therefore, the systematic use of statins in dialysis patients is not currently recommended, due to the lack of observed benefits of this intervention in different studies. However, diabetic patients on dialysis continue to receive this drug due to the extrapolation of the proven benefits of statins in the diabetic population in general.
DR (diabetic retinopathy) is a microvascular complication that can occur in type 1 and type 2 diabetic patients, and its prevalence is closely related to the duration of the disease. The prevalence of this complication increases with the duration of DM, affecting more than 60 % of patients with DM2 and more than 90 % of patients with type 1 DM after 20 years of illness [ 87 ]. DR is the most frequent cause of blindness in adults aged 20–74 years. The pathogenesis of DR is directly linked to chronic hyperglycemia, and diabetic kidney disease is an important factor for an increased risk of DR incidence. DR and diabetic nephropathy are the two most common microvascular complications in patients with DM; however, whether these complications are only related or directly affect each other, or if their progression necessarily occurs simultaneously, is unclear [ 88 ].
Diabetic patients can eventually develop proteinuria, without the presence of DR, or might proliferative DR without the presence of albuminuria. Klein et al. studied a group of normoalbuminuric patients with type 1 DM and found that 36 % of these individuals did not develop DR, 53 % had nonproliferative DR, 9 % had moderate to severe DR, and 2 % had severe DR [ 89 ]. On the other hand, the prevalence of DR in patients with diabetic nephropathy and macroalbuminuria is between 70 and 90 %. Proliferative retinopathy is already considered a predictive factor for macroalbuminuria in type 1 diabetic patients. Some authors consider both microalbuminuria and DR to be predictor factors for the progressive loss of kidney function [ 90 ].
ADA recommends periodic fundus examinations for retinopathy to be treated in a timely manner, before it progresses to irreversible vision loss. Examinations should be conducted at least annually and can be conducted more frequently depending on the degree of retinopathy [ 58 ].
Diabetic cardiovascular autonomic neuropathy
Diabetic autonomic neuropathy is a severe complication of DM and is associated with increased morbidity and mortality and decreased quality of life of the patients. Diabetic autonomic neuropathy can affect different systems. Diabetic cardiovascular autonomic neuropathy (DCAN) can manifest clinically as resting tachycardia, severe orthostatic hypotension, syncope, ischemia and asymptomatic myocardial infarction, systolic and diastolic left ventricular dysfunction, increased risk for CKD, stroke, hyporesponsiveness to hypoglycemia, and sudden cardiac death [ 91 ].
The association between DCAN and kidney disease is also well established and corroborates with the increase in mortality rates in diabetic patients with CKD. Ewing et al. found an upto 53 % increased mortality in diabetic patients with autonomic neuropathy, compared to 15 % in diabetic patients with no dysautonomia. Moreover, half of all deaths in patients with autonomic neuropathy in this study occurred due to impaired renal function, with 29 % of these being sudden death [ 92 , 93 ]. In the literature, the prevalence of autonomic neuropathy varies between 21 and 73 % in the diabetic population. The prevalence of autonomic neuropathy ranges from 20 to 80 % in patients with DKD, and affects 66 % of patients with advanced kidney disease and 50 % of patients on dialysis [ 94 ]. A recent study showed that DCAN presents an important relationship with CKD, albuminuria, and decline in renal function in patients with DM2 [ 95 ].
Treatment of dysautonomic manifestations is essentially symptomatic. Special attention should be given to the intensification of glycemic control, with monitoring of hypoglycemia and changes in lifestyle, including diet and exercise [ 96 ]. Regarding drug treatment, fludrocortisone and the α 1-adrenergic agonist midodrine are considered the drugs of choice in the treatment of DCAN. Erythropoietin is also considered a possible adjunctive drug to increase blood pressure through an increase in the number of erythrocytes and central blood volume, correction of anemia in patients with severe dysautonomia, and neurohumoral effects on wall and vascular tone.
Diabetic genitourinary autonomic neuropathy
Almost half of the patients with DM develop some degree of bladder dysfunction. This prevalence may be even higher in populations with advanced CKD who have DM for a long time, or it may be due to the uremic syndrome per se. Bladder dysfunction might result in varying degrees of impairment, ranging from a mild decrease in bladder sensitivity, reduced emptying perception, and alteration in contractility, to situations where there is an increase in bladder capacity, urinary retention, increased frequency of urinary tract infections, lithiasis, and renal failure [ 97 ].
The prevalence of sexual dysfunction in patients with CKD can range from 9 % in pre-dialysis patients to 70 % in dialysis patients [ 98 ]. In diabetic patients, erectile dysfunction occurs in 35–75 % of patients, 10–15 years earlier than in non-diabetics. In diabetic patients with CKD, the most common causes of erectile dysfunction are organic and are due to vascular disease and neuropathy.
The initial treatment approach for erectile dysfunction in diabetic patients should be glycemic and metabolic control of other associated complications. Specific measures of treatment include drug therapy (group of phosphodiesterase inhibitors: sildenafil, vardenafil, and tadalafil). Intracavernous or intraurethral drugs (papaverine, phentolamine, and prostaglandins) are also used, as well as penile prostheses and vacuum devices [ 96 , 99 ]. However, the use of these drugs requires a more careful evaluation of CKD because of an increased risk of arrhythmias and heart failure.
Nutritional recommendations for diabetic patients with CKD
As diabetic patients experience progressive loss of renal function, nutritional issues become more complex. On one hand, in addition to the existing limitations associated with DM, specific restrictions are needed for patients with CKD, including restriction of protein, phosphorus, and potassium. On the other hand, patients with worsening uremic syndrome have a higher risk of protein-calorie malnutrition that needs to be identified and addressed by the medical team. Thus, nutritional monitoring is of utmost importance in this patient population. Standardized protocols should be avoided, and individualized care and monitoring of patients should be implemented.
Initially, patients should be evaluated based on their standard intake and clinical laboratory results. Then, a nutritional counseling plan should be designed based on nutritional guidelines that aid in the development of appropriate diets for patients, always considering individual needs.
For the population of diabetic patients with CKD in the non-dialytic phase, the composition of macronutrients in the nutritional plan is described in Table 1
For patients with DKD, the ADA (2013) recommends a normoproteic diet (0.8–1.0 g/kg/day) in the early stages of CKD and <0.8 g/kg/day or <0.6 g/kg/day in the later stages of the disease (Table 1 ), with 50 % of protein intake presenting high biological value. Protein restriction aims to act simultaneously as a renoprotective measure, reducing both proteinuria and generation of protein catabolic waste.
For patients with proteinuria >3 g/day, a low protein diet (0.6 g/kg/day) is recommended, as well as the replacement of 1 g of high biological value-protein for each gram excreted. It is important to highlight that provision of a diet low in protein should ensure an adequate energy supply. The recommendation for calorie intake is the same for patients with CKD without the presence of DM—30–35 kcal/kg/day. For overweight and obese patients, the calorie intake recommended should be individualized, although it should not be <25 kcal/kg/day. Regarding glycemic control, the recommended amount of carbohydrates follows the recommendations for the general population (Table 1 ). The quantity and quality of carbohydrates in the diet and its effects on glycemic responses are well established. Sucrose, when consumed in amounts equivalent to that of other carbohydrates, increases blood glucose level equivalently; therefore, sucrose can be consumed in a nutritionally healthy diet as long as its intake does not exceed 10 % of daily caloric consumption. The use of sweeteners, although indicated, is not essential for the treatment of DM. The use of sweeteners can provide beneficial effects, such as weight loss in overweight or obese patients, due to their low caloric value, thus also reducing insulin.
In addition, adequate intake of food rich in complex carbohydrates (dietary fibers) should be encouraged, since this consumption is associated with glycemic control, satiety, and lipid absorption, thus also contributing to weight control. Although consumption of dietary fibers, especially in the soluble fraction, should be encouraged, it is important to highlight that, in general, foods rich in this nutrient fruits, vegetables, and legumes—are also sources of potassium, a mineral for which intake should be controlled in patients with CKD. Main sources of dietary fibers with low potassium levels are fruits such as pineapple, apple, pear and strawberry, and vegetables such as carrot, watercress, lettuce, escarole, cucumber and cabbage.
One method that can be used to control blood glucose in these patients is carbohydrate counting, in which grams of this macronutrient obtained from meals are recorded throughout the day. This method is efficient in food control and the use of insulin, and its orientation should be individualized.
The recommendations for lipid consumption in diabetic patients are the same as those for individuals with cardiovascular diseases (Table 1 ), since both patients are at high risk for cardiovascular events. According to the Guidelines of the SBD (2014), the goals for lipid control in diabetic nephropathy include serum LDL cholesterol levels <100 or <70 mg/dL in the presence of any cardiovascular disease and levels of serum triglycerides <150 mg/dL and high-density lipoprotein cholesterol >40 mg/dL for men and >50 mg/dL for women.
In an interesting study conducted by Cardenas et al. (2004) [ 100 ], it was identified that, in patients with DM with different degrees of renal disease, a greater intake of polyunsaturated fatty acids and lower intake of saturated fatty acids, as well as a higher ratio of unsaturated and saturated fatty acids, promoted a better evolution of diabetic nephropathy. In the same study, it was found that patients with worsening symptoms consumed higher amounts of saturated fatty acids during the 7-year follow-up.
Considering that arterial hypertension is a factor for the progression of diabetic nephropathy, blood pressure control is essential for the treatment of the disease. The American Dietetic Association (2013) recommends a sodium intake <1500 mg/day, which corresponds to 3.75 g/day of salt. In a study conducted by Houlihan et al. (2012) [ 101 ], a diet with 1.2–1.7 g of sodium promoted similar effects to the inclusion of a second antihypertensive drug in the treatment of hypertension.
Pharmacological treatment: non-insulin antidiabetic agents
Control of blood glucose levels in diabetic patients with CKD in different stages is not adequately standardized. Due to the increased risk of hypoglycemia [ 102 ] in these patients, insulin has been considered the safest antidiabetic agent. However, new non-insulin antidiabetic agents proved to be safe and effective. New revisions and guidelines are being published to guide the glycemic control of patients with CKD [ 70 , 103 , 104 ]. Regarding the therapeutic goals, the benefits of strict control of blood glucose levels in recently diagnosed diabetic patients [ 105 ] is not observed in diabetic patients with the disease for a long time and who have already developed cardiovascular complications [ 60 , 63 , 106 ], typical of diabetic patients with CKD. For example, the ACCORD trial noted an increase in the overall mortality of 22 % in diabetic patients with the disease for an average of 10 years with a history of cardiovascular diseases, who received intensive glucose control, and aimed for HbA1c of 6.5 % compared to that in the control group, which was to seeking to achieve HbA1c of 7.3 %. This is explained by the increased risk of hypoglycemia episodes associated with a more intensive management of DM and the fact that these patients are more susceptible to the deleterious effects of hypoglycemia, such as activation of the sympathetic nervous system. However, there are benefits of controlling blood glucose levels in diabetic patients with CKD in terms of reducing mortality rates [ 107 ], inhibiting progression of CKD [ 107 , 108 ], and diminishing albuminuria [ 108 ]. Nevertheless, the therapeutic goals should be individualized and it should be considered that HbA1c overestimates glycemic control in patients with CKD [ 109 ]. Below we discuss several aspects related to CDK of noninsulin antidiabetic agents that are not available in our environment.
Metformin acts primarily in the liver, decreasing the production of hepatic glucose. Therefore, it is associated with low risk of hypoglycemia. This drug has been used for several years and has proven to reduce cardiovascular events [ 105 ] and contribute to mild weight reduction. Thus, it is considered the first choice drug in the treatment of DM2 [ 110 ]. This drug is excreted by the kidney and therefore, in patients with CKD, it may accumulate and increase the risk of lactic acidosis, which is a side effect of this drug. Hence, several authors do not recommend the use of metformin in women with creatinine levels >1.4 mg/dL and men with levels >1.5 mg/dL [ 111 ]. Others recommend halving the dose in patients with a creatinine clearance of 30–45 mL/min/1.73 m 2 and suspension of the drug in patients with <30 mL/min/1.73 m 2 [ 112 ]. The relationship between metformin accumulation and lactic acidosis is not well documented [ 113 ]. Factors such as acidosis, hypoxia, infection, and dehydration are also associated with the advent of lactic acidosis in patients receiving metformin, and in these situations, the drug should be suspended temporarily.
Sulfonylureas
Sulfonylureas act in pancreatic β-cells, releasing insulin. The effectiveness of the class depends on the stores of β-cells, which decreases with the length of the DM. The action of these drugs is independent of glucose levels. Therefore, hypoglycemic episodes are more severe and frequent with the use of sulfonylureas [ 114 ]. In patients with CKD, the use of short-acting sulfonylureas metabolized in the liver, including glipizide, gliclazide, and glimepiride, is recommended. However, the use of this class should be avoided in patients with creatinine clearance <45 mL/min/1.73 m 2 . Sulfonylureas can bind to proteins and are not eliminated by dialysis.
Similarly, glinides, such as repaglinide and nateglinide, act in pancreatic β-cells, releasing insulin. However, these drugs have a shorter half-life and cause less hypoglycemia [ 115 ]. Glinides are metabolized predominantly in the liver. These drugs should be used three times a day before meals and can be used in patients with a creatinine clearance <30 mL/min/1.73 m 2 , although with care and a reduced dosage [ 103 ].
Glitazones, such as pioglitazone and rosiglitazone, increase insulin sensitivity in muscle and adipose tissues by acting on PPAR-ɣ receptors. These drugs are metabolized in the liver, do not accumulate in CKD, and do not cause hypoglycemia, even in patients undergoing dialysis. They are associated with water and salt retention, which limits the use of this class in CKD. It has been shown that the use of rosiglitazone is associated with an increased risk of myocardial infarction [ 116 ] and increased cardiovascular mortality in patients undergoing hemodialysis [ 117 ]. Therefore, pioglitazone has been used more frequently. Glitazones are also associated with a higher risk of fractures and bladder cancer. Despite the low risk of hypoglycemia, this class of drugs should be avoided in patients with CKD.
Alpha-glucosidase inhibitors
Acarbose acts in the gut by inhibiting alpha-glucosidase, the enzyme responsible for digesting carbohydrates. It does not cause hypoglycemia. Its main side effect is flatulence. In CKD, its use should be avoided, since it accumulates and can cause hepatotoxicity [ 118 ].
Sodium-glucose cotransporter type 2 inhibitors
In the glomeruli, about 180 g of glucose per day is filtered, and nearly all is reabsorbed in the S1 segment of the proximal tubule by sodium-glucose cotransporters. Of these, type 2 cotransporters are the most important [ 119 ]. Drugs that inhibit this transporter have been developed, such as dapagliflozin, canagliflozin, and empagliflozin. These drugs block reabsorption of glucose and sodium in the proximal tubule, contributing to improved glycemic control, with no risk of hypoglycemia, as well as hypertension control, due to increased natriuresis. The use of these drugs is associated with a higher incidence of genital infection. This hypoglycemic class is not indicated in patients with a creatinine clearance <45 mL/min/1.73 m 2 [ 120 ], but recent studies show a potential application in the lower (30 ml/min) GFR range. Recent data suggests cardiovascular benefits of this class, opening opportunities for a broader application of SGLT-inhibitors [ 120 ].
Peptide-1 receptor agonists similar to glucagon (GLP-1 RA)
GLP-1 is an incretin secreted in the gastrointestinal tract in response to food intake. It acts on pancreatic β-cells, releasing insulin, and in pancreatic α-cells, inhibiting the secretion of glucagon in a glucose-dependent manner; therefore, GLP-1 controls blood glucose with a lower risk of hypoglycemia. Moreover, it slows gastric emptying and decreases appetite through a central mechanism, thus contributing to weight loss. GLP-1 receptor agonists, such as exenatide and liraglutide, are peptides with a structure similar to endogenous GLP-1. However, these drugs are resistant to enzyme dipeptidyl peptidase-4 catabolism. The route of administration is subcutaneous. Since they are peptides, they are filtered in the glomeruli and degraded in the proximal tubules, similar to the process associated with insulin. There is little knowledge regarding this class of antidiabetic drugs in CKD, although gastrointestinal effects are exacerbated in patients with CKD, including nausea, vomiting, and diarrhea. Moreover, there have been reported cases of acute renal injury with the use of exenatide in patients with CKD [ 121 ]. Therefore, while acquiring further knowledge about this class, it is suggested that careful attention be paid to their use in patients with creatinine clearance 45–60 mL/min/1.73 m 2 . Also, its use should be avoided in patients with a creatinine clearance <45 mL/min/1.73 m 2 [ 103 ].
Dipeptidyl peptidase-4 (DPP-4) inhibitors
DPP-4 is an enzyme that degrades GLP-1 and GIP incretins. Therefore, DPP-4 inhibitors increase the concentrations of GLP-1 and GIP, which, as mentioned above, act in pancreatic β-cells by releasing insulin, and in pancreatic α-cells, inhibiting the secretion of glucagon in a glucose-dependent manner, thus controlling blood glucose with no risk of hypoglycemia. The greatest effect of DPP-4 inhibitors is in the postprandial period, when the levels of glucose are elevated. DPP-4 inhibitors are also known as gliptins. Four gliptins are available: vildagliptin, sitagliptin, saxagliptin, and linagliptin. This antidiabetic class is becoming more important among diabetic patients with CKD, due to their excellent tolerability profile [ 122 – 126 ]. Vildagliptin, sitagliptin, and saxagliptin are excreted by the kidney and require dosage adjustment in patients with creatinine clearance <50 mL/min/1.73 m 2 . For example, vildagliptin, which is used at a dosage of 50 mg twice a day, should be used at the same dose, but as a single daily administration in patients with creatinine clearance <50 mL/min/1.73 m 2 , including patients with Stage 5 CKD [ 124 ]. Linagliptin has no renal excretion and therefore does not require adjustment for renal function.
Until recently, the arsenal of noninsulin antidiabetic agents was not safe to be used in diabetic patients with CKD, and insulin therapy was started early, causing psychological distress to patients and families. Nowadays, there are new noninsulin agents, DPP-4 inhibitors in particular, which present a low risk of hypoglycemia and can be used in patients with DM2 with CKD. However, further studies are required to confirm the safety of these new agents in this population. Table 2 summarizes the recommendations for the use of noninsulin antidiabetic agents for noninsulin patients based on international guidelines [ 70 , 103 , 104 ].
Pharmacological treatment of DM in CKD: insulin therapy
The kidney plays an important role in clearing insulin from the systemic circulation and two distinct pathways have been described; one involves glomerular filtration and subsequent insulin absorption by proximal tubular cells through endocytosis; and the other is related to insulin diffusion through peritubular capillaries and their connection to the contraluminal tubular membrane, especially from the distal half of the nephron. Therefore, insulin is transported by lysosomes and metabolized to amino acids that are released by diffusion in peritubular vessels, and final degradation products are then reabsorbed [ 127 – 129 ]. Endogenous insulin has a mean plasma half-life of only 6 min and it is almost cleared from the circulation within 10–15 min (Fig. 3 a). Except for the portion of insulin bound to its receptors on the target cells, the remainder is degraded mainly in the liver, to a lesser extent in kidney and muscle and slightly in most other tissues. In contrast, exogenous insulin does not undergo the first-pass effect in the liver, the kidney plays an important role in the metabolism and clearance of circulating insulin in patients with renal failure (Fig. 3 b). As a consequence, with the progression of CKD, insulin clearance decreases, thus requiring a dose reduction in order to avoid hypoglycemia [ 130 , 131 ].
Adapted from Iglesias and Díez [ 130 ]
Schematic presentation of the clearance of insulin. a endogenous insulin and b exogenous insulin.
The pharmacokinetics of commercially available insulin in diabetic patients with reduced glomerular filtration rate has been evaluated for small number of studies. Although the profile of these patients requires less insulin, several authors suggest a reduction of the dose of insulin when GFR is between 10–50 mL/min, around 25 % of total daily dose and 50 % for a GFR <10 mL/min, regardless of the type of insulin used [ 132 ].
Insulins are classified according to their action profile (Table 3 ). Thus, the first exogenous insulins developed to control blood sugar, NPH (Neutral Protamine Hagedorn) and Regular insulin are labeled as having an intermediate- and rapid-acting profile, respectively. One has a peak activity 4–7 h after subcutaneous injection, while the other one is used before meals in order to reduce the peak of hyperglycemia after the ingestion of carbohydrates. However, its onset of action is between 30 min and 1 h and it must be applied around 30–45 min before the meal. The insulin analogs, produced by recombinant DNA technology, are classified as (1) short-acting (lispro, aspart, and glulisine insulin), (2) long-acting (glargine, detemir), or (3) ultra-long-acting (degludec). The association between the short-acting and the long- or ultra-long-acting insulin analogs enables physiological simulation of insulin secretion; this therapeutic association has been termed basal-bolus insulinization.
Due to its pharmacokinetic profile with a stable half-life and duration of action of about 24 h, glargine insulin can be prescribed once a day. To date, few studies have been published on the use of glargine insulin in patients with renal failure, and its use appears to be safe, with a reduction in HbA1c in a short period of time [ 133 ]. In hospitalized patients with a GFR <45 mL/min, the reduction of the dosage calculated according to the body weight was shown to be effective in diminishing the number of hypoglycemic events by 50 %, without compromising metabolic control [ 134 ]. Detemir insulin has an onset of drug action of 1 h, and its effect lasts 12–24 h. Thus, it is recommended that this drug be used in two daily doses, with intervals of about 12 h. However, some patients could present different sensitivity along the day, and for this subgroup of patients a single-a-day dose may be enough to maintaining adequate glycemic control in the postprandial period [ 135 , 136 ]. A recent study [ 137 ] demonstrated the need for dose reduction, for both glargine and detemir insulin, in patients with renal function impairment. In this case, the dose of glargine and detemir insulin was 29.7 and 27.3 % lower in individuals with GFR <60 mL/min than in those with GFR >90 mL/min. Degludec insulin, with an ultra-long-action profile, has recently been approved to be commercialized, and only one study in patients with different stages of renal failure and terminal CKD has been published, showing no statistical significant differences in absorption or release profiles when compared to individuals with normal renal function. Thus, degludec insulin does not require dose adjustments due to the loss of kidney function [ 138 ].
As shown on Table 3 , the insulin analogs lispro, aspart, and glulisine have short durations and very similar pharmacokinetic profiles [ 139 ]. Because lispro insulin was the first analog investigated, there are a number of studies in patients with CKD [ 140 – 142 ] showing it has a beneficial effect in reducing glomerular hyperfiltration and renal effects of hyperglycemia triggered by meals; these effects are possibly related to an antagonistic effect on insulin-like growth factor-1 [ 140 ]. Furthermore, the use of lispro insulin was associated with improved glycemic control and quality of life in patients on hemodialysis by end-stage diabetic renal disease [ 141 , 142 ]. The glulisine and aspart insulin also had their safety and efficacy demonstrated in controlling postprandial hyperglycemia in patients with DM2 and severe renal failure [ 143 ]. No change in the pharmacokinetic of these drugs was observed [ 144 ].
Regardless of insulin being considered the best choice for glycemic control in patients with renal impairment, its prescription must be based on some guidelines, such as: (1) individualization of the therapy; (2) frequent reassessment of prescription or adjustment of doses for the glomerular filtration rate; (3) basal-bolus insulin regimens, prescribing intermediate- or long-acting profile insulin, as basal insulin, to keep the levels of blood glucose stable on post-absorptive period, associated with short-acting profile insulin to promote adequate carbohydrates metabolism and control of postprandial glycaemia; and (4) blood glucose monitoring and frequent adjustment of insulin therapy based on individual response [ 145 ]. Few studies have reported specific information on the differences in action profiles, half-life, metabolism, and clearance of different insulin types available that are adjusted for the different stages of CKD; such studies would allow the prescription of more effective therapeutic regimens, minimizing risk of hypoglycemia, which is potentially more harmful in this population. Therefore, the treatment should be individualized based on factors such as the presence of complications, associated diseases, disease management ability, stage and duration of CKD, and previous glycemic control [ 146 – 148 ]. In addition, there should be participation of a multidisciplinary team consisting of nephrologists, endocrinologists, nutritionists, and nurses. This approach has proved to be an effective strategy in achieving individual glycemic optimal values, reducing the rate of progression of kidney disease and other complications associated with DM2, and improving the quality of life of patients with DKD.
The relationship between DM and DKD is more complicated than the predisposition of a diabetic patient to develop kidney disease and the negative impact on morbidity and mortality of patients with kidney disease and DM. Recently, the kidney has been recognized as being directly involved in the pathogenesis of DM because of its ability to regulate glucose reabsorption as well as to determine insulin half-life and resistance. In addition, it is now clear that glomerular filtration provides a safe and efficacious target for many hypoglycemic drugs. Thus, understanding the renal physiology and pathophysiology of DKD has become essential to all specialties treating diabetic patients. Disseminating this knowledge and detailing the evidence will be important to initiate breakthrough research and to encourage proper treatment of this group of patients.
Abbreviations
diabetes mellitus
diabetic kidney disease
diabetes mellitus type 2
chronic kidney disease
insulin resistance
glucose transporters of the cell membrane
glucose transporter of the cell membrane 4
homeostatic model assessment for insulin resistance
glucose-dependent insulinotropic peptide
glucagon-like peptide-1
sodium/glucose cotransporter
sodium/glucose cotransporter 1
sodium/glucose cotransporter 2
United Kingdom Prospective Diabetes Study
hemoglobin A1c
home glucose monitoring or self-monitoring
hemoglobin A
national glycohemoglobin standardization program
high performance liquid chromatography
diabetes control and complications trial
ethylene diamine tetraacetic acid
hemoglobin C
hemoglobin D
hemoglobin E
hemoglobin S
glycated albumin
1,5-anhydroglucitol
Brazilian Diabetes Society
American Diabetes Society
action to control cardiovascular risk in diabetes
action in diabetes and vascular disease
glomerular filtration rate
National Kidney Foundation-Kidney Disease Outcomes Quality Initiative
angiotensin-converting enzyme
angiotensin II receptor blockers
Veterans Affairs Nephropathy in Diabetes
kidney disease: improving global outcomes
low-density lipoprotein cholesterol
diabetic retinopathy
diabetic cardiovascular autonomic neuropathy
peroxisome proliferator-activated receptor ɣ
glucagon-like peptide-1 receptor agonist
dipeptidyl peptidase-4
American Diabetes Association. Standards of care in diabetes 2014. Diabetes Care. 2014;37:S14–80.
Article Google Scholar
DeFronzo Ralph. From the triumvirate to the ominous octet: a new paradigm for the treatment of type 2 diabetes mellitus. Diabetes. 2009;58:773–95.
Article CAS PubMed PubMed Central Google Scholar
Porte D. Central regulation of energy homeostasis. Diabetes. 2006;55(Suppl 2):S155–60.
Article CAS Google Scholar
Schwartz MW, Woods SC, Porte D, Seeley RJ, Baskin DC. Central nervous system control of food intake. Nature. 2000;404:661–71.
CAS PubMed Google Scholar
Plum L, Belgardt BF, Bruning JC. Central insulin action in energy and glucose homeostasis. J Clin Invest. 2006;116:1761–6.
Bonadonna RC, Groop L, Kraemer N, Ferrannini E, Del Prato S, DeFronzo RA. Obesity and insulin resistance in humans: a dose-response study. Metabolism. 1990;39:452–9.
Article CAS PubMed Google Scholar
Shulman GI. Cellular mechanisms of insulin resistance. J Clin Invest. 2000;106:171–6.
Parente EB, Pereira PHGR, Nunes VS, Lottenberg AMP, Lima CSLM, Rochitte CE, et al. The effects of high-fat or high-carbohydrate diet on intramyocellular lipids. J Food Nutr Disord. 2014;3:6.
Google Scholar
DeFronzo RA, Tobin JD, Andres R. Glucose clamp technique: a method for quantifying insulin secretion and resistance. Am J Physiol Endocrinol Metab Gastrointest Physiol. 1979;237:E214–23.
CAS Google Scholar
Matthews DR, Hosker JP, Rudenski AS, Naylor BA, Treacher DF, Turner RC. Homeostasis model assessment: insulin resistance and beta-cell function from fasting plasma glucose and insulin concentration in man. Diabetologia. 1985;28:412–9.
Matsuda M, DeFronzo RA. Insulin sensitivity indices obtained from oral glucose tolerance testing: comparison with the euglycemic insulin clamp. Diabetes Care. 1999;22:1462–70.
Perley MJ, Kipnis DM. Plasma insulin responses to oral and intravenous glucose: studies in normal and diabetic subjects. J Clin Invest. 1967;46:1954–62.
Druker DJ. Enhancing incretin action for the treatment of type 2 diabetes. Diabetes Care. 2003;26:2929–40.
Ozaki N, Shibasaki T, Kashima Y, Miki T, Takahashi K, Ueno H, et al. cAMP-GEFII is a direct target of cAMP in regulated exocytosis. Nat Cell Biol. 2000;2:805–11.
Sivertsen J, Rosenmeier J, Holst JJ, Vilsbøll T. The effect of glucagon-like peptide 1 on cardiovascular risk. Nat Rev Cardiol. 2012;9:209–22.
Bergman H, Drury DR. The relationship of kidney function to the glucose utilization of the extra abdominal tissues. Am J Physiol. 1938;124:279–84.
Meriel P, Galinier F, Suc J, et al. Le metabolisme duRein humaian. Rev Franc Etudes Clin Biol. 1958;3:332–4.
Nieth H, Schollmeyer P. Substrate utilization of the human kidney. Nature. 1966;209:1244–5.
Björkman O, Felig P. Role of the kidney in the metabolism of fructose in 60-h fasted humans. Diabetes. 1982;31:516–20.
Article PubMed Google Scholar
Aber G, Morris L, Housley E. Gluconeogenesis by the human kidney. Nature. 1966;212:1589–90.
Meyer C, Dostou J, Nadkarni V, Gerich J. Effects of physiological hyperinsulinemia on systemic, renal and hepatic substrate metabolism. Am J Physiol. 1998;275:F915–21.
Cersosimo E, Garlick P, Ferretti J. Insulin regulation of renal glucose metabolism in humans. Am J Physiol. 1999;276:E78–84.
Stumvoll M, Chintalapudi U, Perriello G, Welle S, Gutierrez O, Gerich J. Uptake and release of glucose by the human kidney: postabsorptive rates and responses to epinephrine. J Clin Invest. 1995;96:2528–33.
Stumvoll M, Meyer C, Kreider M, Perriello G, Gerich J. Effects of glucagon on renal and hepatic glutamine gluconeogenesis in normal postabsorptive humans. Metabolism. 1998;47:1227–32.
Schoolwerth A, Smith B, Culpepper R. Renal gluconeogenesis. Miner Electrolyte Metab. 1988;14:347–61.
Wirthensohn G, Guder W. Renal substrate metabolism. Physiol Rev. 1986;66:469–97.
Meyer C, Gerich JE. Role of the kidney in hyperglycemia in type 2 diabetes. Curr Diab Rep. 2002;2:237–41.
Abe M, Kalantar-Zadeh K. Hemodialysis induced hypoglycemia and glucose disarrays. Nat Rev Nephrol. 2015;11:302–13.
U.S. Renal Data System. Incidence, prevalence, patient characteristics and treatment modalities. National Institutes of Health, National Institute of Diabetes and Kidney Diseases. In: USRDS 2011 Annual data report. Bethesda; 2011.
UK Prospective Diabetes Study Group. Effect of intensive blood glucose control with metformin on complications in overweight patients with type 2 diabetes: UKPDS 34. Lancet. 1998;352:854–65.
Koenig RJ, Peterson CM, Jones RL, et al. Correlation of glucose regulation and hemoglobin AIc in diabetes mellitus. N Engl J Med. 1976;295:417–20.
Herman WH, Cohen RM. Racial and ethnic differences in the relationship between HbA1c and blood glucose: implications for the diagnosis of diabetes. J Clin Endocrinol Metab. 2012;97:1067–72.
The American Diabetes Association, European Association for the Study of Diabetes, International Federation of Clinical Chemistry and Laboratory Medicine, International Diabetes Federation. Consensus statement on the worldwide standardization of the HbA1c measurement. Diabetologia. 2007;50:2042–3.
Kilpatrick ES, Rigby AS, Atkin SL. Variability in the relationship between mean plasma glucose and HbA1c: implications for the assessment of glycemic control. Clin Chem. 2007;53:897–901.
Miller WG, Myers GL, Ashwood ER, Killeen AA, Wang E, Ehlers GW, et al. State of the art in trueness and interlaboratory harmonization for ten analytes in general clinical chemistry. Arch Pathol Lab Med. 2008;132:838–46.
Selvin E, Crainiceanu CM, Brancati FL, Coresh J. Short-term variability in measures of glycemia and implications for the classification of diabetes. Arch Intern Med. 2007;167:1545–51.
Pimazoni-Netto A, Rodbard D, Zanella MT. Rapid improvement of glycemic control in type 2 diabetes using weekly intensive multifactorial interventions: structured glucose monitoring, patient education, and adjustment of therapy—a randomized controlled trial. Diabetes Technol Ther. 2011;13(10):997–1004. doi: 10.1089/dia.2011.0054 .
Article PubMed PubMed Central Google Scholar
Nathan D, Davidson MB, Defronzo RA, Heine RJ, Henry RR, Pratley R, American Diabetes Association, et al. Impaired fasting glucose and impaired glucose tolerance: implications for care. Diabetes Care. 2007;30:753–9.
Lenters-Westra E, Schindhelm RK, Bilo HJ, et al. Haemoglobin A1c: historical overview and current concepts. Diabetes Res Clin Pract. 2013;99:75–84.
Chen HS, Wu TE, Lin HD, Jap TS, Hsiao LC, Lee SH, et al. Hemoglobin A(1c) and fructosamine for assessing glycemic control in diabetic patients with CKD stages 3 and 4. Am J Kidney Dis. 2010;55:867–74.
Little RR, Rohlfing CL, Wiedmeyer HM, Myers GL, Sacks DB, Goldstein DE. The national glycohemoglobin standardization program: a 5-year progress report. Clin Chem. 2001;47:1985–92.
Gallagher EJ, Le Roith D, Bloomgarden Z. Review of hemoglobin A(1c) in the management of diabetes. J Diabetes. 2009;1:9–17.
Uzu T, Hatta T, Deji N, Izumiya T, Ueda H, Miyazawa I, et al. Target for glycemic control in type 2 diabetic patients on hemodialysis: effects of anemia and erythropoietin injection on hemoglobin A(1c). Ther Apher Dial. 2009;13:89–94.
Speeckaert M, Biesen WV, Delanghe J, Slingerland R, Wiecek A, Heaf J, The European Renal Best Practice Guideline Development Group on Diabetes in Advanced CKD, et al. Are there better alternatives than haemoglobin A1c to estimate glycaemic control in the chronic kidney disease population? Nephrol Dial Transplant. 2014;0:1–11.
Mackenzie T, Brooks B, O’Connor G. Beverage intake, diabetes, and glucose control of adults in America. Ann Epidemiol. 2006;16:688–91.
Okada T, Nakao T, Matsumoto H, Yamanaka T, Nagaoka Y, Tamekuni T. Influence of age and nutritional status on glycated albumin values in hemodialysis patients. Intern Med. 2009;48:1495–9.
Cohen RM, Holmes YR, Chenier TC, Joiner CH. Discordance between HbA1c and fructosamine: evidence for a glycosylation gap and its relation to diabetic nephropathy. Diabetes Care. 2003;26:163–7.
Mittman N, Desiraju B, Fazil I, Kapupara H, Chattopadhyay J, Jani CM, et al. Serum fructosamine versus glycosylated hemoglobin as an index of glycemic control, hospitalization, and infection in diabetic hemodialysis patients. Kidney Int Suppl. 2010;117:S41–5.
Yamanouchi T, Akanuma Y. Serum 1,5-anhydroglucitol (1,5 AG): new clinical marker for glycemic control. Diabetes Res Clin Pract. 1994;24:S261–8.
Nerby CL, Stickle DF. 1,5-anhydroglucitol monitoring in diabetes: a mass balance perspective. Clin Biochem. 2009;42:158–67.
Ricks J, Molnar MZ, Kovesdy CP, Shah A, Nissenson AR, Williams M, et al. Glycemic control and cardiovascular mortality in hemodialysis patients with diabetes: a 6-year cohort study. Diabetes. 2012;61:708–15.
Hill CJ, Maxwell AP, Cardwell CR, Freedman BI, Tonelli M, Emoto M, et al. Glycated hemoglobin and risk of death in diabetic patients treated with hemodialysis: a meta-analysis. Am J Kidney Dis. 2014;63:84–94.
Spiegel DM, Breyer JA. Serum albumin: a predictor of long-term outcome in peritoneal dialysis patients. Am J Kidney Dis. 1994;23:283–5.
Freedman BI. A critical evaluation of glycated protein parameters in advanced nephropathy: a matter of life or death: time to dispense with the hemoglobin A1C in end-stage kidney disease. Diabetes Care. 2012;35:1621–4.
Little RR, Rohlfing CL, Tennill AL, Hanson SE, Connolly S, Higgins T, et al. Measurement of Hba(1C) in patients with chronic renal failure. Clin Chim Acta. 2013;418:73–6.
UK Prospective Diabetes Study. UKPDS) Group. Intensive blood-glucose control with sulphonylureas or insulin compared with conventional treatment and risk of complications in patients with type 2 diabetes (UKPDS 33. Lancet. 1998;352(9131):837–53.
The Diabets Control and Complications Trial Resesarch Group. The effect of intensive treatment of diabetes on the development and progression of long-term complications in insulin-dependent diabetes mellitus. N Engl J Med. 1993;329:977–86.
de Diabetes SB. Diretrizes da Sociedade Brasileira de. Diabetes. 2014;2013–2014:147–65.
Grant RW, Donner TW, Fradkin JE, et al. Professional practice committee for the standards of medical care in diabetes-2015. Diabetes Care. 2015;38(Suppl 1):S88–9. doi: 10.2337/dc15-S018 .
Action to Control Cardiovascular Risk in Diabetes Study Group, Gerstein HC, Miller ME, Byington RP, Goff DC, Bigger JT, et al. Effects of intensive glucose lowering in type 2 diabetes. New Engl J Med. 2008;358:2545–59.
Gosmanov AR, Gosmanova EO. Long-term renal outcomes of patients with type 1 diabetes mellitus and microalbuminuria: an analysis of the DCCT/EDIC cohort. Arch Intern Med. 2011;171(5):412–20.
Perkins BA, Ficociello LH, Silva KH, Finkelstein DM, Warram JH, Krolewski AS. Regression of microalbuminuria in type 1 diabetes. N Engl J Med. 2003;348:2285–93.
ADVANCE Collaborative Group, Patel A, MacMahon S, Chalmers J, Neal B, Billot L, et al. Intensive blood glucose control and vascular outcomes in patients with type 2 diabetes. N Engl J Med. 2008;358(24):2560–72. doi: 10.1056/NEJMoa0802987 .
DeFronzo RA, Alvestrand A, Smith D, Hendler R, Hendler E, Wahren J. Insulin resistance in uremia. J Clin Invest. 1981;67(2):563–8. doi: 10.1172/JCI110067 .
Adrogué HJ. Glucose homeostasis and the kidney. Kidney Int. 1992;42(5):1266–82. doi: 10.1038/ki.1992.414 .
Charpentier G, Riveline JP, Varroud-Vial M. Management of drugs affecting blood glucose in diabetic patients with renal failure. Diabetes Metab. 2000;26(4):73–85. http://www.ncbi.nlm.nih.gov/pubmed/10922977 .
Carone FA, Peterson DR. Hydrolysis and transport of small peptides by the proximal tubule. Am J Physiol. 1980;238(3):F151–8. http://www.ncbi.nlm.nih.gov/pubmed/6989268 .
Mak RH, DeFronzo RA. Glucose and insulin metabolism in uremia. Nephron. 1992;61(4):377–82. http://www.ncbi.nlm.nih.gov/pubmed/1501732 .
Alicic RZ, Tuttle KR. Management of the diabetic patient with advanced chronic kidney disease. Semin Dial. 2010;23(2):140–7. doi: 10.1111/j.1525-139X.2010.00700.x .
Williams ME, Garg R. Glycemic management in ESRD and earlier stages of CKD. Am J Kidney Dis. 2014;63(2 Suppl 2):S22–38. doi: 10.1053/j.ajkd.2013.10.049 .
Amico JA, Klein I. Diabetic management in patients with renal failure. Diabetes Care. 1981;4(3):430–4. http://www.ncbi.nlm.nih.gov/pubmed/10922977 .
Mühlhauser I, Toth G, Sawicki PT, Berger M. Severe hypoglycemia in type I diabetic patients with impaired kidney function. Diabetes Care. 1991;14(4):344–6. http://www.ncbi.nlm.nih.gov/pubmed/2060440 .
Miller CD, Phillips LS, Ziemer DC, Gallina DL, Cook CB, El-Kubbi IM. Hypoglycemia in patients with type 2 diabetes mellitus. Arch Intern Med. 2001;161(13):1653–9. doi: 10.1001/archinte.161.13.1653 .
Levey AS, Eckardt KU, Tsukamoto Y, Levin A, Coresh J, Rossert J, et al. Definition and classification of chronic kidney disease: a position statement from kidney disease: improving global outcomes (KDIGO). Kidney Int. 2005;67(6):2089–100. doi: 10.1111/j.1523-1755.2005.00365.x .
Bode BW, Gross TM, Thornton KR, Mastrototaro JJ. Continuous glucose monitoring used to adjust diabetes therapy improves glycosylated hemoglobin: a pilot study. Diabetes Res Clin Pract. 1999;46(3):183–90. http://www.ncbi.nlm.nih.gov/pubmed/10624783 .
Andrassy KM. Comments on ‘KDIGO 2012 clinical practice guideline for the evaluation and management of chronic kidney disease’. Kidney Int. 2013;84(3):622–3.
ACCORD study group, Cushman WC, Evans GW, Byington RP, Goff DC, Grimm RH, et al. Effects of intensive blood-pressure control in type 2 diabetes mellitus. N Engl J Med. 2010;29(362):1575–85.
Parving H, Hommel E, Smidt UM. Protection of kidney function and decrease in albuminuria by captopril in insulin dependent diabetics with nephropathy. BMJ. 1988;297(6656):1086–91.
James PA, Oparil S, Carter BL, Cushman WC, Dennison-Himmelfarb C, Handler J, et al. 2014 evidence-based guideline for the management of high blood pressure in adults: report from the panel members appointed to the Eighth Joint National Committee (JNC 8). JAMA. 2014;311(5):507–20. doi: 10.1001/jama.2013.284427 .
Fried LF, Emanuele N, Zhang JH, Brophy M, Conner TA, Duckworth W, et al. Combined angiotensin inhibition for the treatment of diabetic nephropathy. N Engl J Med. 2013;369(20):1892–903. doi: 10.1056/NEJMoa1303154 .
PalmerSuetonia C, et al. Comparative efficacy and safety of blood pressure-lowering agents in adults with diabetes and kidney disease: a network meta-analysis. Lancet. 2015;385(9982):2047–56.
Pepine CJ, Handberg EM, Cooper-DeHoff RM, Marks RG, Kowey P, Messerli FH, et al. A calcium antagonist vs a non-calcium antagonist hypertension treatment strategy for patients with coronary artery disease. The International Verapamil-Trandolapril study (INVEST): a randomized controlled trial. JAMA. 2003;290(21):2805–16. doi: 10.1001/jama.290.21.2805 .
Fineberg D, Jandeleit-Dahm KA, Cooper ME. Diabetic nephropathy: diagnosis and treatment. Nat Rev Endocrinol. 2013;9(12):713–23.
KDIGO. Clinical practice guideline for lipid management in chronic kidney disease. Kidney Inter. 2013;3(3):259–305.
Sarnak MJ, Bloom R, Muntner P, Rahman M, Saland JM, Wilson PW, et al. KDOQI US commentary on the 2013 KDIGO clinical practice guideline for lipid management in CKD. Am J Kidney Dis. 2015;65(3):354–66. doi: 10.1053/j.ajkd.2014.10.005 .
American Diabetes Association. Standards of medical care in diabetes—2014. Diabetes Care. 2014;37(Suppl 1):S14–80. doi: 10.2337/dc14-S014 .
Krentz AJ, Clough G, Byrne CD. Interactions between microvascular and macrovascular disease in diabetes: pathophysiology and therapeutic implications. Diabetes Obes Metab. 2007;9(6):781–91. doi: 10.1111/j.1463-1326.2007.00670.x .
Moriya T, Tanaka S, Kawasaki R, Ohashi Y, Akanuma Y, Yamada N, Japan Diabetes Complications Study, et al. Diabetic retinopathy and microalbuminuria can predict macroalbuminuria and renal function decline in Japanese type 2 diabetic patients. Diabetes Care. 2013;36(9):2803–9. doi: 10.2337/dc12-2327 .
Klein R, Zinman B, Gardiner R, Suissa S, Donnellly SM, Sinaiko AR, et al. The relationship of diabetic retinopathy to preclinical diabetic glomerulopathy lesions in type 1 diabetic patients. Diabetes. 2005;54(2):527–33.
Tong PCY, Kong AP, So WY, Yang X, Ng MCY, Ho CS, et al. Interactive effect of retinopathy and macroalbuminuria on all-cause mortality, cardiovascular and renal end points in Chinese patients with type 2 diabetes mellitus. Diabet Med. 2007;24(7):741–6. doi: 10.1111/j.1464-5491.2007.02145.x .
Joy MS, Cefalu WT, Hogan SL, Nachman PH. Long-term glycemic control measurements in diabetic patients receiving hemodialysis. Am J Kidney Dis. 2002;39(2):297–307. doi: 10.1053/ajkd.2002.30549 .
Ewing DJ, Campbell IW, Clarke BF. The natural history of diabetic autonomic neuropathy. Q J Med. 1980;49(193):95–108.
Spallone V, Ziegler D, Freeman R, Bernardi L, Frontoni S, Pop-Busui R, et al. Cardiovascular autonomic neuropathy in diabetes: clinical impact, assessment, diagnosis, and management. Diabetes Metab Res Rev. 2011;27(7):639–53. doi: 10.1002/dmrr.1239 .
Tahrani AA, Dubb K, Raymond NT, Begum S, Altaf QA, Sadiqi H, et al. Cardiac autonomic neuropathy predicts renal function decline in patients with type 2 diabetes: a cohort study. Diabetologia. 2014;57(6):1249–56. doi: 10.1007/s00125-014-3211-2 .
National Kidney Foundation. KDOQI clinical practice guideline for diabetes and CKD: 2012 update. Am J Kidney Dis. 2012;60(5):850–86. doi: 10.1053/j.ajkd.2012.07.005 .
Foss-Freitas MC, MarquesJunior W, Foss MC. Autonomic neuropathy: a high risk complication for type 1 diabetes mellitus. Arq Bras Endrocrinol Metab. 2008;52(2):398–406.
Arrellano-Valdez F, Urrutia-Osorio M, Arroyo C, Soto-Vega E. A comprehensive review of urologic complications in patients with diabetes. Springerplus. 2014;3:549. doi: 10.1186/2193-1801-3-549 .
Procci WR, Goldstein DA, Adelstein J, Massry SG. Sexual dysfunction in the male patient with uremia: a reappraisal. Kidney Int. 1981;19(2):317–23.
Vinik AI, Maser RE, Mitchell BD, Freeman R. Diabetic autonomic neuropathy. Diabetes Care. 2003;26(5):1553–79.
Cárdenas C, Bordiu E, Bagazoitia J, Calle-Pascual AL, Diabetes and Nutrition Study Group, Spanish Diabetes Association. Polyunsaturated fatty acid consumption may play role in the onset and regression of microalbuminúria in well-controlled type 1 and type 2 diabetic people: a 7-year, prospective, population-based, observational multicenter study. Diabetes Care. 2004;27(6):1454–7.
Houlihan CA, Allen TJ, Baxter AL, Panangiotopoulos S, Casley DJ, Cooper ME, et al. A low-sodium diet potentiates the effects of losartan in type 2 diabetes. Diabetes Care. 2002;25(4):663–71.
Moen MF, Zhan M, Hsu VD, Walker LD, Einhorn LM, Seliger SL, et al. Frequency of hypoglycemia and its significance in chronic kidney disease. Clin J Am Soc Nephrol. 2009;4(6):1121–7.
Gómez-Huelgas R, Martínez-Castelao A, Artola S, Górriz JL, Menéndez E. Consensus document on treatment of type 2 diabetes in patients with chronic kidney diseases. Nefrologia. 2014;34(1):34–45.
PubMed Google Scholar
Garg R, Williams ME. Diabetes management in the kidney patient. Med Clin North Am. 2013;97(1):135–56.
Stratton IM, Adler AI, Neil HA, Matthews DR, Manley SE, Cull CA, et al. Association of glycaemia with macrovascular and microvascular complications of type 2 diabetes (UKPDS 35): prospective observational study. BMJ. 2000;321(7258):405–12.
Duckworth W, Abraira C, Moritz T, Reda D, Emanuele N, Reaven PD, et al. Glucose control and vascular complications in veterans with type 2 diabetes. N Engl J Med. 2009;360(2):129–39.
Shurraw S, Hemmelgarn B, Lin M, Sr Majumdar, Klarenbach S, Manns B, et al. Association between glycemic control and adverse outcomes in people with diabetes mellitus and chronic kidney disease: a population-based cohort study. Arch Intern Med. 2011;171(21):1920–7.
Lachin JM, Viberti G, Zinman B, Haffner SM, Aftring RP, Paul G, et al. Renal function in type 2 diabetes with rosiglitazone, metformin, and glyburide monotherapy. Clin J Am Soc Nephrol. 2011;6(5):1032–40.
Little RR, Tennill AL, Rohlfing C, Wiedmeyer HM, Khanna R, Goel S, et al. Can glycohemoglobin be used to assess glycemic control in patients with chronic renal failure? Clin Chem. 2002;48(5):784–6.
American Diabetes Association. Standards of medical care in diabetes—2012. Diabetes Care. 2012;35(Suppl 1):S11–63.
Runge S, Mayerle J, Warnke C, Robinson D, Roser M, Felix SB, et al. Metformin-associated lactic acidosis in patients with renal impairment solely due to drug accumulation? Diabetes Obes Metab. 2008;10(1):91–3.
Lipska KJ, Bailey CJ, Inzucchi SE. Use of metformin in the setting of mild-tomoderate renal insufficiency. Diabetes Care. 2011;34:1431–7.
Nye HJ, Herrington WG. Metformin: the safest hypoglycaemic agent in chronic kidney disease? Nephron Clin Pract. 2011;118:c380–3.
Holstein A, Plaschke A, Hammer C, Egberts EH. Characteristics and time course of severe glimepiride-versus glibenclamide induced hypoglycaemia. Eur J Clin Pharmacol. 2003;59(2):91–7.
Hasslacher C, Multinational Repaglinide Renal Study Group. Safety and efficacy of repaglinide in type 2 diabetic patients with and without impaired renal function. Diabetes Care. 2003;26:886–91.
Nissen SE, Wolski K. Effect of rosiglitazone on the risk of myocardial infarction and death from cardiovascular causes. N Engl J Med. 2007;356(24):2457–71.
Ramirez SP, Albert JM, Blayney MJ, Tentori F, Goodkin DA, Wolfe RA, et al. Rosiglitazone is associated with mortality in chronic hemodialysis patients. J Am Soc Nephrol. 2009;20(5):1094–101.
Hsiao SH, Liao LH, Cheng PN, Wu TJ. Hepatotoxicity associated with acarbose therapy. Ann Pharmacother. 2006;40(1):151–4.
Mather A, Pollock C. Glucose handling by the kidney. Kidney Int Suppl. 2011;120:S1–6.
Shyangdan DS, Uthman OA, Waugh N. SGLT-2 receptor inhibitors for treating patients with type 2 diabetes mellitus: a systematic review and network meta-analysis. BMJ Open. 2016;6(2):9417.
Dubois-Laforgue D, Boutboul D, Lévy DJ, Joly D, Timsit J. Severe acute renal failure in patients treated with glucagon-like peptide-1 receptor agonists. Diabetes Res Clin Pract. 2014;103(3):e53–5.
Chan JC, Scott R, Arjona Ferreira JC, Sheng D, Gonzalez E, Davies MJ, et al. Safety and efficacy of sitagliptin in patients with type 2 diabetes and chronic renal insufficiency. Diabetes Obes Metab. 2008;10(7):545–55.
Graefe-Mody U, Friedrich C, Port A, Ring A, Retlich S, Heise T, et al. Effect of renal impairment on the pharmacokinetics of the dipeptidyl peptidase-4 inhibitor linagliptin(*). Diabetes Obes Metab. 2011;13(10):939–46.
Lukashevich V, Schweizer A, Shao Q, Groop PH, Kothny W. Safety and efficacy of vildagliptin versus placebo in patients with type 2 diabetes and moderate or severe renal impairment: a prospective 24-week randomized placebo-controlled trial. Diabetes Obes Metab. 2011;13(10):947–54.
Nowicki M, Rychlik I, Haller H, Warren M, Suchower L, gause-Nilsson I, et al. Long-term treatment with the dipeptidyl peptidase-4 inhibitor saxagliptin in patients with type 2 diabetes mellitus and renal impairment: a randomised controlled 52-week efficacy and safety study. Int J Clin Pract. 2011;65(12):1230–9.
Nowicki M, Rychlik I, Haller H, Warren ML, Suchower L, Gause-Nilsson I, D1680C00007 Investigators. Saxagliptin improves glycaemic control and is well tolerated in patients with type 2 diabetes mellitus and renal impairment. Diabetes Obes Metab. 2011;13(6):523–32.
Rabkin R, Ryan MP, Duckworth WC. The renal metabolism of insulin. Diabetologia. 1984;27(3):351–7.
Duckworth WC, Kitabchi AE. Insulin metabolism and degradation. Endocr Rev. 1981;2(2):210–33.
Duckworth WC. Insulin degradation: mechanisms, products, and significance. Endocr Rev. 1988;9(3):319–45.
Iglesias P, Díez JJ. Insulin therapy in renal disease. Diabetes Obes Metab. 2008;10(10):811–23.
Charlesworth JA, Kriketos AD, Jones JE, Erlich JH, Campbell LV, Peake PW. Insulin resistance and postprandial triglyceride levels in primary renal disease. Metabolism. 2005;54(6):821–8.
Freeman JS. Review of insulin-dependent and insulin-independent agents for treating patients with type 2 diabetes mellitus and potential role for sodium-glucose co-transporter 2 inhibitors. Postgrad Med. 2013;125(3):214–26.
Niafar M, Nakhjavani M. Efficacy and safety of insulin glargine in type 2 diabetic patients with renal failure. J Diabetes Metab. 2012;03(04):1–4.
Baldwin D, Lee H, Zander J, Emanuele MA, Munoz C, Glossop V, et al. A randomized trial of two weight-based doses of insulin glargine and glulisine in hospitalized subjects with type 2 diabetes and renal insufficiency. Diabetes Care. 2012;35:1970–4.
Jones MC, Patel M. Insulin detemir: a long-acting insulin product. Am J Health Syst Pharm. 2006;63(24):2466–72.
Porcellati F, Rossetti P, Busciantella NR, Marzotti S, Lucidi P, Luzio S, et al. Comparison of pharmacokinetics and dynamics of the long-acting insulin analogs glargine and detemir at steady state in type 1 diabetes: a double-blind, randomized, crossover study. Diabetes Care. 2007;30:2447–52.
Kulozik F, Hasslacher C. Insulin requirements in patients with diabetes and declining kidney function: differences between insulin analogues and human insulin? Ther Adv Endocrinol Metab. 2013;4(4):113–21.
Kiss I, Arold G, Roepstorff C, Bøttcher SG, Klim S, Haahr H. Insulin degludec: pharmacokinetics in patients with renal impairment. Clin Pharmacokinet. 2014;53(2):175–83.
Bode BW. Use of rapid-acting insulin analogues in the treatment of patients with type 1 and type 2 diabetes mellitus: insulin pump therapy versus multiple daily injections. Clin Ther. 2007;29:135–44.
Ruggenenti P, Flores C, Aros C, Ene-Iordache B, Trevisan R, Ottomano C, et al. Renal and metabolic effects of insulin lispro in type 2 diabetic subjects with overt nephropathy. Diabetes Care. 2003;26(2):502–9.
Ersoy A, Ersoy C, Altinay T. Insulin analogue usage in a haemodialysis patient with type 2 diabetes mellitus. Nephrol Dial Transplant. 2006;21(2):553–4.
Czock D, Aisenpreis U, Rasche FM, Jehle PM. Pharmacokinetics and pharmacodynamics of lispro-insulin in hemodialysis patients with diabetes mellitus. Int J Clin Pharmacol Ther. 2003;41(10):492–7.
Urata H, Mori K, Emoto M, Yamazaki Y, Motoyama K, Morioka T, et al. Advantage of insulin glulisine over regular insulin in patients with type 2 diabetes and severe renal insufficiency. J Ren Nutr. 2015;25(2):129–34.
Holmes G, Galitz L, Hu P, Lyness W. Pharmacokinetics of insulin aspart in obesity, renal impairment, or hepatic impairment. Br J Clin Pharmacol. 2005;60(5):469–76.
Lubowsky ND, Siegel R, Pittas AG. Management of glycemia in patients with diabetes mellitus and CKD. Am J Kidney Dis. 2007;50(5):865–79.
Cefalu WT. Diabetes care: “state of the union”. Diabetes Care. 2012;36(1):1–3.
Article PubMed Central Google Scholar
Ramirez SPB, McCullough KP, Thumma JR, Nelson RG, Morgenstern H, Gillespie BW, et al. Hemoglobin A(1c) levels and mortality in the diabetic hemodialysis population: findings from the Dialysis Outcomes and practice patterns study (DOPPS). Diabetes Care. 2012;35(12):2527–32.
Rhee CM, Leung AM, Kovesdy CP, Lynch KE, Brent GA, Kalantar-Zadeh K. Updates on the management of diabetes in dialysis patients. Semin Dial. 2014;27(2):135–45.
Download references
Authors’ contributions
All authors contributed reviewing the literature and writing the manuscript. RPF and SV did the final review and editing. All authors made substantial contributions to conception and design, or acquisition of data, or analysis and interpretation of data; had been involved in drafting the manuscript or revising it critically for important intellectual content and had given final approval of the version to be published. All the authors agreed to be accountable for all aspects of the work in ensuring that questions related to the accuracy or integrity of any part of the work are appropriately investigated and resolved. All authors read and approved the final manuscript.
Acknowledgements
The authors would like to thank Editage ( www.editage.com.br ) for English language editing. The authors would also like to thank Maria Kamimura for all her assistance during the discussions and preparation of the manuscript.
Competing interests
The authors declare that they have no competing interests.
Availability of data and materials
Not applicable.
Consent for publication
Ethics approval and consent to participate.
Our workgroup formed by nephrologists, endocrinologists and diabetologists worked between 2014 and 2015 discussing issues related to diabetes and the kidney were sponsored by Novartis in a complete independent educational program.
Author information
Authors and affiliations.
School of Medicine, Pontificia Universidade Católica do Paraná, Imaculada Conceição, 1155, Curitiba, PR, 80215-901, Brazil
Roberto Pecoits-Filho
School of Medicine, University of São Paulo, São Paulo, Brazil
Hugo Abensur, Alisson Diego Machado, Márcia Queiroz & Silvia Titan
Hospital Regional de Presidente Prudente, Universidade do Oeste Paulista, Presidente Prudente, São Paulo, Brazil
Carolina C. R. Betônico
Santa Casa de São Paulo, São Paulo, Brazil
Erika B. Parente & João Eduardo Nunes Salles
Institute of Pharmaceutical Sciences, Goiania, Brazil
Sergio Vencio
You can also search for this author in PubMed Google Scholar
Corresponding author
Correspondence to Roberto Pecoits-Filho .
Rights and permissions
Open Access This article is distributed under the terms of the Creative Commons Attribution 4.0 International License ( http://creativecommons.org/licenses/by/4.0/ ), which permits unrestricted use, distribution, and reproduction in any medium, provided you give appropriate credit to the original author(s) and the source, provide a link to the Creative Commons license, and indicate if changes were made. The Creative Commons Public Domain Dedication waiver ( http://creativecommons.org/publicdomain/zero/1.0/ ) applies to the data made available in this article, unless otherwise stated.
Reprints and permissions
About this article
Cite this article.
Pecoits-Filho, R., Abensur, H., Betônico, C.C.R. et al. Interactions between kidney disease and diabetes: dangerous liaisons. Diabetol Metab Syndr 8 , 50 (2016). https://doi.org/10.1186/s13098-016-0159-z
Download citation
Received : 09 May 2016
Accepted : 10 July 2016
Published : 28 July 2016
DOI : https://doi.org/10.1186/s13098-016-0159-z
Share this article
Anyone you share the following link with will be able to read this content:
Sorry, a shareable link is not currently available for this article.
Provided by the Springer Nature SharedIt content-sharing initiative
- Type 2 diabetes
- Diabetic kidney disease
- Diabetes complications
- Glycemic control
Diabetology & Metabolic Syndrome
ISSN: 1758-5996
- Submission enquiries: [email protected]
Home — Essay Samples — Nursing & Health — Kidney — Kidney dialysis

Kidney Dialysis
- Categories: Kidney
About this sample

Words: 557 |
Published: Mar 1, 2019
Words: 557 | Page: 1 | 3 min read
Works Cited:
- Committee on the Rights of the Child. (2007). General comment No. 11: Indigenous children and their rights under the Convention.
- Committee on the Rights of the Child. (2011). General comment No. 13: The right of the child to freedom from all forms of violence.
- Committee on the Rights of the Child. (2013). General comment No. 17: The right of the child to rest, leisure, play, recreational activities, cultural life and the arts (art. 31).
- Committee on the Rights of the Child. (2014). General comment No. 15: The right of the child to the enjoyment of the highest attainable standard of health (art. 24).
- Committee on the Rights of the Child. (2016). General comment No. 20: On the implementation of the rights of the child during adolescence.
- Committee on the Rights of the Child. (2017). General comment No. 19: Public budgeting for the realization of children’s rights (art. 4).
- Committee on the Rights of the Child. (2019). General comment No. 24: Children’s rights in the child justice system.
- Committee on the Rights of the Child. (2020). General comment No. 18: On harmful practices.
- Convention on the Rights of the Child. (1989). United Nations Treaty Collection.
- Office of the High Commissioner for Human Rights. (2003). International human rights standards for law enforcement: A pocket book on human rights for the police.
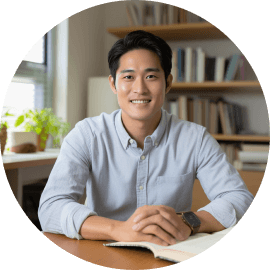
Cite this Essay
Let us write you an essay from scratch
- 450+ experts on 30 subjects ready to help
- Custom essay delivered in as few as 3 hours
Get high-quality help

Dr Jacklynne
Verified writer
- Expert in: Nursing & Health
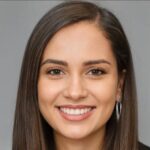
+ 120 experts online
By clicking “Check Writers’ Offers”, you agree to our terms of service and privacy policy . We’ll occasionally send you promo and account related email
No need to pay just yet!
Related Essays
2 pages / 1119 words
1 pages / 668 words
1 pages / 616 words
3 pages / 1510 words
Remember! This is just a sample.
You can get your custom paper by one of our expert writers.
121 writers online
Still can’t find what you need?
Browse our vast selection of original essay samples, each expertly formatted and styled
Related Essays on Kidney
This experiment is to analyze kidney filtration by using simple filtration system. In this experiment, the protein, glucose and starch were used as tested substances. The procedure is repeated as the first experiment was as [...]
Kidney Disease is a disease affecting the kidneys and its functions. There are many different forms of kidney disease and various outcomes. In order to recognize kidney disease, there are many symptoms that someone would be able [...]
Addiction has an enormous impact on the people lives especially in the manner in which it affects their lives. Furthermore, this also affects their families since it has the tendencies of putting the relationships at risks due [...]
The Immune system is the body’s overall defense system against harm causing invaders, that come from the outside world, that are able to inter the body one way or another. The immune system is not an organ or two that do all [...]
The ileum is the longest segment of the small intestine and it contains a circular smooth muscular layer. The smooth muscle contains muscarinic acetylcholine receptors which should respond to the presence of acetylcholine (ACh) [...]
The esophagus or gullet is a muscular canal, about 23 to 25 cm. long, extending from the pharynx to the stomach. It begins in the neck at the lower border of the cricoids cartilage, opposite the sixth cervical vertebra, descends [...]
Related Topics
By clicking “Send”, you agree to our Terms of service and Privacy statement . We will occasionally send you account related emails.
Where do you want us to send this sample?
By clicking “Continue”, you agree to our terms of service and privacy policy.
Be careful. This essay is not unique
This essay was donated by a student and is likely to have been used and submitted before
Download this Sample
Free samples may contain mistakes and not unique parts
Sorry, we could not paraphrase this essay. Our professional writers can rewrite it and get you a unique paper.
Please check your inbox.
We can write you a custom essay that will follow your exact instructions and meet the deadlines. Let's fix your grades together!
Get Your Personalized Essay in 3 Hours or Less!
We use cookies to personalyze your web-site experience. By continuing we’ll assume you board with our cookie policy .
- Instructions Followed To The Letter
- Deadlines Met At Every Stage
- Unique And Plagiarism Free

An official website of the United States government
The .gov means it’s official. Federal government websites often end in .gov or .mil. Before sharing sensitive information, make sure you’re on a federal government site.
The site is secure. The https:// ensures that you are connecting to the official website and that any information you provide is encrypted and transmitted securely.
- Publications
- Account settings
Preview improvements coming to the PMC website in October 2024. Learn More or Try it out now .
- Advanced Search
- Journal List
- Endocr Connect
- v.10(5); 2021 May 1
Chronic kidney disease in patients with diabetes mellitus
Espen nordheim.
1 Department of Transplantation Medicine, Section of Nephrology, Oslo University Hospital, Rikshospitalet, Oslo, Norway
2 Faculty of Medicine, University of Oslo, Oslo, Norway
Trond Geir Jenssen
3 Metabolic and Renal Research Group, Faculty of Health Sciences, UiT- The Arctic University of Norway, Tromsø

Chronic kidney disease is a common complication and concomitant condition of diabetes mellitus. The treatment of patients with diabetes and chronic kidney disease, including intensive control of blood sugar and blood pressure, has been very similar for type 1 and type 2 diabetes patients. New therapeutic targets have shown promising results and may lead to more specific treatment options for patients with type 1 and type 2 diabetes.
Introduction
Diabetes mellitus is among the leading causes of chronic kidney disease and end-stage kidney disease in the western world. It was the most common diagnosis for the initiation of renal replacement therapy in the United States in 2018, accounting for 47% of the cases ( 1 ).
Type 1 and type 2 diabetes mellitus share many clinical characteristics and long-term complications, but they are in fact two different diseases with diverging pathophysiology ( 2 ). While type 1 diabetes results from autoimmune destruction of the insulin-producing beta-cells within the pancreatic islets of Langerhans, type 2 diabetes is most often characterized by insulin resistance together with insufficient insulin response, at least in White people.
Type 1 and type 2 diabetes mellitus can both cause long-term microvascular and macrovascular complications, contributing to the increased morbidity and mortality among these patients. Kidney disease in patients with diabetes can be a result of microvascular complications from diabetes, a concomitant kidney disease of other origin or a combination of the two. In type 1 diabetes patients, microvascular disease secondary to diabetes is the most common etiology to chronic kidney disease, while a spectrum of etiologies can cause kidney disease in type 2 diabetes patients.
The established treatment principals to prevent and halt progression of chronic kidney disease have been very similar in the two diabetic phenotypes. This review article intends to highlight the established and upcoming treatment for patients with type 1 and type 2 diabetes and chronic kidney disease. We have reviewed landmark papers published up till the fall of 2020 and publications dealing with new potential therapeutic targets. This review is based on literature search in Pubmed with the following combinations of keywords: 'diabetic nephropathy', 'diabetic kidney disease', 'diabetic nephropathy treatment' and 'diabetic kidney disease treatment' to complete our own bibliography. The search yielded a total of 79.367 search results and was closed on October 26, 2020. The relevance of the articles was initially assessed on the basis of title and abstract before relevant articles in English language were read in full text. Reference lists of all articles read were analyzed in order to identify reference articles for the review.
Kidney disease in patients with type 1 diabetes mellitus
Type 1 diabetes usually affects young and middle-aged patients and among these patients, chronic kidney disease is most often caused by diabetes-related microvascular disease ( 3 ), a condition which has been referred to as diabetic nephropathy or 'diabetic kidney disease' in the literature.
Chronic kidney disease in type 1 diabetes patients typically can cause a progressive decline in renal function. Hyperglycaemia starts the pathophysiologic mechanisms with a subsequent interplay of altered haemodynamics, metabolic and inflammatory pathways ( 4 ). When glomerular foot processes merge and the integrity of the glomerular basement membrane is compromised by pathological processes, albumin and subsequently, larger proteins can leak into the urine. Urine albumin/creatinine ratio (UACR) spot analysis of first morning collection is preferred for proteinuria quantification, above assessment of albumin in 24 h urine collection. UACR are staged into moderate albuminuria, previously called microalbuminuria (30–300 mg/g) and overt albuminuria, previously called proteinuria (≥300 mg/g).
Chronic kidney disease in type 1 diabetes patients is initially characterized by hyperfiltration due to increased glomerular filtration pressure ( 5 ). Cherney et al . postulated hyperglycaemia-dependent hyperfiltration to be mediated through upregulated back-transportation of sodium and glucose from the renal tubular system ( 6 ). Sodium-glucose-co-transporter-2 (SGLT2) contributes to 90% of this transportation reducing distal tubular flux of glucose and sodium. Due to reduced sodium flux in the loop of Henle, macula densa signals dilatation of the afferent arteriolar tone through a tubuloglomerular feedback mechanism which increases tubular sodium flux at the expense of increase of intraglomerular pressure and hyperfiltration at the nephron level. Hyperfiltration is in the clinic seen as an increase in glomerular filtration rate (GFR) ( 5 , 6 ). Albuminuria and hypertension subsequently occur as the kidney disease develops. After the initial hyperfiltration phase, nephrons are lost resulting in a steady GFR decline ranging 3–6 mL/min/year ( 4 ). Renal failure requiring replacement therapy may eventually occur within 20–25 years. During this process, the remaining nephrons compensate by hyperfiltration not only due to hyperglycemia but now also due to reduced total filtration surface. This represents a vicious circle with progressive loss of nephrons.
In his first model of the disease, Mogensen suggested that the pathological processes in chronic kidney disease in type 1 diabetes patients were irreversible and that the disease gradually progressed ( 5 ). Later, this model has been challenged by reports describing that renal function may be reduced without concomitant proteinuria and that proteinuria may cease spontaneously in the course of the disease ( 7 ). These reports used estimated GFR based on cystatin c measurements, and they have, thus, been challenged since these estimates of GFR may differ substantially from measured GFR ( 8 ).
Kidney disease in patients with type 2 diabetes mellitus
While chronic kidney disease in type 1 diabetes most often is secondary to diabetes microvascular disease, there is a whole spectrum of chronic kidney disease etiologies in type 2 diabetes. Type 2 diabetes patients are often older at the time of diagnosis and kidney disease due to other causes than diabetes is likely to occur. Several studies have verified that kidney disease in type 2 diabetes may be a more compounded entity than what is seen in type 1 diabetes ( 9 ). One study from the United States which examined kidney biopsies in patients with type 2 diabetes and kidney disease found that typical diabetic microvascular disease were present in 37% of the cases, non-diabetic kidney disease in 36% of the cases, such as nephrosclerosis or immunological kidney disease, while mixed forms of diabetic and non-diabetic kidney disease were found in 27% of the cases ( 10 ). Interestingly, one study has found different insulin resistance phenotypes in diabetes to be associated with different risks for chronic kidney disease ( 11 ), but this needs to be studied further.
Regardless of kidney disease etiology, strict blood glucose control is on a group level the single-most important intervention to prevent kidney disease to develop in patients with type 1 and type 2 diabetes ( 12 , 13 , 14 ). Normalization of blood glucose might act renoprotective through different mechanisms: reduced hyperfiltration on the nephron level ( 15 , 6 ), reduced generation of toxic intermediates such as reactive oxygen species (ROS) ( 16 ) and reduced activity in pathogenetic signalling pathways including the polyol, hexasamine, protein kinase C and advanced glycation end-product pathways ( 17 ).
Still as the chronic kidney disease progresses, GFR is reduced through nephron loss and hyperfiltration in remaining nephrons drives the process further.
How to diagnose kidney disease in patients with diabetes?
Typically, patients with established diabetes are diagnosed with kidney disease when albuminuria is identified as elevated in two out of three spot urine analyzes, corresponding to urine albumin/creatinine ratio (UACR) at or above 30 mg/g and/or persistently impaired renal function, defined as estimated glomerular filtration rate (eGFR) below 60 mL/1.73 m 2 .
Our clinical impression is that, in most cases, patients with diabetes are not referred for diagnostic kidney biopsy when kidney disease is verified, especially if the clinical characteristics do not differ from what can be expected from chronic kidney disease in diabetes (i.e. increased albuminuria, hypertension and slow decrease in estimated GFR). However, other diseases must be suspected when no other microvascular complications of diabetes are present and also in the presence of microscopic haematuria ( 9 ). The pathological classification system developed for chronic kidney disease in type 1 diabetes also includes type 2 diabetes ( 3 ). It has been argued that a common classification system is challenging due to the differences in renal lesions among the two diseases ( 18 ). While type 1 diabetes patients typically have glomerular lesions characterized by thickening of the glomerular basement membrane, mesangial expansion and glomerulosclerosis, only 30% of type 2 diabetes patients with microalbuminuria and 50% with proteinuria demonstrate such lesions ( 19 ).
Established treatment of chronic kidney disease for type 1 and type 2 diabetes
Intensive blood sugar control.
Long-term hyperglycaemia causes glomerular hyperfiltration together with glycation of cell proteins, especially intranuclar proteins and nucleic acids. Intensified blood sugar control reduces the risk for proteinuria to develop and also to progress ( 12 , 13 , 14 ). Prevention of overt proteinuria is beneficial for maintaining renal function over time ( 12 , 13 , 14 ). The effect is well-documented in patients with type 1 diabetes ( 12 , 13 ) and has also been demonstrated at a group level for patients with type 2 diabetes ( 14 ). Most guidelines recommend glucose control corresponding to HbA1c around 53 mmol/mol (7%), provided good quality of life is maintained without causing repeated or even severe episodes of hypoglycaemia ( 20 , 21 ).
The risk for severe hypoglycaemic episodes increases when GFR falls below 45 mL/min. Hypoglycaemic episodes may develop due to reduced gluconeogenesis and counter-regulation in the kidneys ( 22 ) and also the half-life of blood glucose-lowering drugs can be prolonged when renal function is impaired, which may necessitate dose-lowering or cessation of drugs such as insulin or sulfonylureas. The increased risk for metformin-related lactacidosis must also been taken into consideration when renal function is impaired ( 23 ).
Regulation of blood pressure and albuminuria is beneficial for chronic kidney disease in diabetes
Carl Erik Mogensen reported in 1976 on how blood pressure must be lowered in order to prevent decline in renal function in patients with type 1 diabetes ( 24 ). The treatment target for this patient group is blood pressure below 130/80 mmHg ( 25 , 26 ). Blocking of the renin-angiotensin-aldosterone (RAAS) system is particularly effective in order to reduce proteinuria and preserve renal function, regardless of the blood pressure, first demonstrated for captopril in patients with type 1 diabetes and nephropathy ( 27 ) and later for losartan and irbesartan in type 2 diabetes ( 28 , 29 ). Angiotensin-converting enzyme inhibitors (ACEi) and angiotensin II receptor blockers (ARBs) reduce intraglomerular filtration pressure by dilating the efferent glomerular arteriole, and the clinical effects of these two classes of drugs are considered as equivalent ( 30 ). If proteins are present in the urine, it is crucial to reduce the level of proteinuria in order to reduce the risk for kidney disease progression ( 31 ). To achieve a reduction of proteinuria, RAAS-blocking drugs are often needed in higher doses to reduce proteinuria than for reaching the blood pressure target alone ( 32 ). To be able to monitor and evaluate treatment effect, the amount of proteinuria should be quantified and followed with ACR in urine, which can be easily analyzed in spot samples of morning urine ( 33 ).
Other interventions beneficial for chronic kidney disease in diabetes
A comprehensive care is recommended for the management of diabetes patients in the recent KDIGO clinical practice guidelines ( 21 ), including statins to all patients with diabetes and kidney disease regardless of cholesterol levels to prevent cardiovascular disease. Moderate physical activity for at least 150 min per week is recommended in addition to smoking cessation, weight loss in the case of obesity, salt restriction and protein-reduced diet.
Recent studies on glucose-lowering treatment with clinical impact for patients with type 2 diabetes
Patients with type 1 diabetes need treatment with exogenous insulin to obtain blood glucose control, while type 2 diabetes patients might reach treatment targets with life-style modifications, with or without addition of oral glucose lowering agents. The last decade has presented a large selection of blood-glucose lowering drugs for type 2 diabetes patients, and it has been demonstrated that especially two classes of drugs have particular beneficial effects in patients with kidney disease beyond the blood-glucose lowering effect: receptor agonists of the glucagon-like protein 1 receptor (GLP-1 RA) and inhibitors of sodium-glucose-co-transporter-2 (SGLT2i). In secondary analyses of the SAVOR-TIMI 53 and CARMELINA studies, the dipeptidyl peptidase-4 inhibitors (DPP4i) – saxagliptin and linagliptin were associated with less development of macroalbuminuria ( 34 , 35 ) but with no effect on major cardiovascular endpoints ( 36 , 35 ). However, the antiproteinuric effect is more pronounced with GLP-1 RAs. Renal outcomes were included in six out of the major GLP-1 RAs studies on cardiovascular outcome (CVOT): lixisenatide (ELIXA), liraglutide (LEADER), semaglutide (SUSTAIN-6), exenatide (EXSCEL), dulaglutide (REWIND and AWARD-7) ( 37 , 38 , 39 , 40 , 41 , 42 ). Major adverse cardiovascular events and death were the primary outcomes of all these studies, while composite renal endpoints were among the secondary outcomes. The renal endpoints were not standardized, but all included proteinuria and change in estimated GFR. Lixisenatide, liraglutide, semaglutide, exenatide and dulaglutide all seemed to demonstrate a protective effect on composite renal outcomes. This effect was apparently driven by reduced development of macroalbuminuria and not by stabilization of GFR ( 37 , 38 , 40 ). However, dulaglutide seemed to preserve renal function better compared to insulin glargine after 1 year of treatment in patients with type 2 diabetes in AWARD-7 ( 42 ).
How GLP-1 RAs provide beneficial effects in the kidneys, outside lowering of blood glucose, is not fully understood. It has been suggested that GLP-1 agonism mediates reduced inflammation and oxidative stress. GLP-1 RA increases natriuresis, probably by inhibiting sodium/hydrogen isoform 3 (NHE3) in the renal tubules ( 43 ), but the renal haemodynamic effects seem neutral as the drug also causes glomerular vasodilatation ( 44 ). Weight loss, another known effect of GLP-1 RA leading to reduced hyperfiltration and improved proteinuria, might also have a role.
Promising results were found with the SGLT2-inhibitors early in the cardiovascular safety studies, for example, with empagliflozin (EMPA-REG), canagliflozin (CANVAS) and dapagliflozin (DECLARE- TIMI 58) ( 45 , 46 , 47 , 48 ). Composite renal outcomes were studied as secondary endpoints also in these studies, and it was observed that SGLT2i not only seemed to reduce proteinuria but also to delay deterioration of renal function (eGFR).
How can we understand the SGLT2i effects in chronic kidney disease?
SGLT2-mediated retention of glucose in the kidneys is probably a calorie saving mechanism which enabled survival in times when access to exogenous energy resources were more restricted than today. In a normal diet, the kidneys reabsorb 180 g glucose (720 kilocalories) daily. During diabetes and hyperglycaemia, the reabsorption of glucose and sodium is increased even more and less sodium is presented to macular densa distal to loop of Henle. This in turn initiates a tubuloglomerular feedback mechanism which dilates the afferent arteriole leading to an increase in GFR. Thus, tubular sodium flux is reestablished at the expense of hyperfiltration which is detrimental for the glomerular tuft in the long run.
The excretion of sodium into the urine increases due to the co-inhibitory effect of SGLT2i, which again normalizes the afferent arteriole tone through its effect on macula densa ( 6 ). Increased afferent arteriole tone translates into a reduced filtration pressure in the glomeruli and, thus, reduced load on the glomerular tuft. Additional potential beneficial mechanisms of SGLT2i may be increased oxygenation of tubular cells, possible reduction of toxic effects on renal tubules secondary to reduced albuminuria, preservation of intravascular volume and reduced volume overload due to loop-diuretic sparing effect in addition to improved metabolic parameters (i.e. body weight and HbA1c) ( 49 ). One study found post-glomerular vasodilatation of the SGLT2 inhibitor dapagliflozin rather than pre-glomerular vasoconstriction in metformin-treated patients with type 2 diabetes ( 50 ).
The CREDENCE study was the first study that primarily included type 2 diabetes patients with kidney disease ( 51 ). This was a randomized placebo-controlled trial of more than 4000 type 2 diabetes patients with estimated glomerular filtration rate (eGFR) between 30 and 90 mL/min/1.73 m 2 and UACR ranging 300–5000 mg/g. The pre-specified efficacy criteria for the study were achieved earlier than expected and led to an early termination of the study ( https://www.jnj.com/phase-3-credence-renal-outcomes-trial-of-invokana-canagliflozin-is-being-stopped-early-for-positive-efficacy-findings ; accessed October 26, 2020). After a median follow-up of 2.6 years, the treatment group had a significant reduction in the combined renal-specific endpoint (development of terminal kidney failure, doubling of serum creatinine or death from renal-specific cause). These results were valid also for the patients with the most reduced renal function in the study. In fact, in a subset of patients with a GFR drop to less than 30 mL/min/1.73 m 2 just prior to randomization, safety and efficacy of the drug was in line with the whole study population ( 52 ). Renoprotective effects occur at GFR thresholds where glucose lowering is no longer observed. The ability for SGLT2i to lower blood glucose decreases sharply when estimated GFR falls below 60 mL/min/1.73 m 2 ( 53 ), while the CREDENCE study documented kidney protective effects in the patient group with estimated GFR ranging from 30 to 45 mL/min/1.73 m 2 and even lower.
The DAPA-CKD study studied whether the SGLT2 inhibitor dapagliflozin could have beneficial renal effects also in non-diabetic patients with chronic kidney disease (CKD). Due to superior effects on the renal endpoints, the study was halted before scheduled time ( 54 ). The study involved 4.304 patients with CKD out of which only 68% had type 2 diabetes, and the primary end-point was: decline in the estimated GFR of at least 50%, end-stage kidney disease, or death from renal or cardiovascular causes. Patients with eGFR down to 25 mL/min/1.73 m 2 were included. The study found significant reduction in the primary endpoint and the effect was found independently of presence of diabetes. The number of patients needed to be treated to prevent one incident was as low as 19 during follow-up (median 2.4 years). The EMPEROR-Reduce study, published simultaneously, studied the effect of empagliflozin in heart failure patients already on RAAS blockage and found that empagliflozin was superior to placebo in preventing cardiovascular death or hospitalization for heart failure ( 55 ). Rate of eGFR decline was a secondary outcome of the study. The annual decline in eGFR was slower in the empagliflozin group compared to the placebo group (0.55 mL/min/1.73 m 2 vs 2.28 mL/min/1.73 m 2 ). The DAPA-HF trial studied the effect of dapagliflozin in heart failure patients and found a lower risk of heart failure worsening or death from cardiovascular causes in the treatment group compared to placebo, regardless of the presence of absence of diabetes ( 56 ). The incidence of the prespecified renal composite outcome did not differ between the treatment groups. The number of patients with kidney disease in the DAPA-HF trial was low which may have reduced the power to detect statistically significant differences.
The VERTIS study could not demonstrate a significant effect of ertugliflozin on the primary major adverse cardiovascular events endpoint, but the results showed numerically beneficial outcome for preservation of GFR compared to placebo ( 57 ). Table 1 summarizes the recent SGLT2i studies with demonstrated effects on renal endpoint.
Summary of recent SGLT2i studies with effects on primary and secondary renal endpoints.
The ongoing EMPA-KIDNEY study also addresses the renal outcome of using the SGLT2 inhibitor empagliflozin in persons with CKD. In this study also patients with type 1 diabetes are included, in addition to patients with type 2 diabetes or no diabetes (ClinicalTrials.gov. {"type":"clinical-trial","attrs":{"text":"NCT03594110","term_id":"NCT03594110"}} NCT03594110 ). The study plans to recruit in total 5000 patients including type 1 diabetes, type 2 diabetes and non-diabetic patients with impaired renal function (eGFR ≥ 20 to <45 mL/min/1.73 m 2 or eGFR ≥ 45 to <90 mL/min/1.73 m 2 and UACR ≥ 200 mg/g) and the results are estimated to be published by summer 2022.
In the 2020 KDIGO guidelines for diabetes management in chronic kidney disease, the combination of metformin and SGLT2i is now recommended as first line treatment for all type 2 diabetes patients with chronic kidney disease if eGFR is above 30 mL/min per 1.73 m 2 regardless of glucose control, even in patients with HbA1c within the target range. If the patient does not achieve the individualized glycaemic target, the addition of a GLP-1 RA is recommended ( 21 ). Previous and upcoming studies on SGLT2-inhibitors studies are all performed in patients already treated with RAAS blockage or inhibition. Thus, one might expect that current treatment with ACEi or ARBs will still be a basic therapy in the future.
Other therapeutic targets recently tested
Atrasentan, an endothelin A-receptor antagonist, was investigated in the SONAR study ( 58 ). Atrasentan is thought to have beneficial effects in several pathophysiological processes involved in chronic kidney disease in patients with diabetes, altered haemodynamics, inflammation and fibrosis. The study included type 2 diabetes patients with eGFR 25–75 mL/min/1.73 m 2 and UACR 300–5000 mg/g and demonstrated a lower reduction in eGFR and reduced proteinuria when compared to placebo. Another and smaller clinical study on pirfenidion, an inhibitor of TGF-β (transforming grown factor beta), included patients with both type 1 and type 2 diabetes with kidney failure and proteinuria and reported improvement in eGFR after 1 year treatment compared to placebo ( 59 ). Sulodexide, a glycosaminoglycan described as being able to prevent structural changes in the glomerular basement membrane, has shown positive effects on proteinuria in two small studies ( 60 , 61 ). Bardoxolone mathyl is demonstrated to improve inulin GFR in a Japanese phase 2 study, the phase 3 study results are expected early in 2022 ( 62 ). Lowering of the serum urate level with allopurinol was ,however, not shown to slow the decrease in the glomerular filtration rate (GFR) in persons with type 1 diabetes and early-to-moderate diabetic nephropathy ( 63 ). FIDELIO-DKD found promising results with finerenone, a selective mineralocorticoid, on diabetic nephropathy and cardiovascular endpoints ( 64 ).
Studies in progress
In addition to the EMPA-KIDNEY study which examines SGLT2i in type 1 diabetes patients, other studies are of potential interest. The FLOW trial (Semaglutide on the progression of renal impairment in subjects with type 2 diabetes and chronic kidney disease) is designed to show whether the GLP-1 RA semaglutide can slow the decline in eGFR among type 1 diabetes patients with chronic kidney disease. The trial is still recruiting patients and will evaluate when a sufficient amount of end-points has been obtained, alternatively after 5 years. The primary endpoint is persistent eGFR decline of >50%, reaching end-stage renal disease, death from kidney disease or death from cardiovascular disease (ClinicalTrials.gov identifier: {"type":"clinical-trial","attrs":{"text":"NCT03819153","term_id":"NCT03819153"}} NCT03819153 ).
FIGARO-DKD will give us more answers to the role of finerenone in the treatment of chronic kidney disease in type 1 diabetes patients and cardiovascular endpoints (ClinicalTrials.gov: {"type":"clinical-trial","attrs":{"text":"NCT02545049","term_id":"NCT02545049"}} NCT02545049 ). Another interesting study investigates whether reduced oxidative stress by inhibiting NADPH oxidase can reduce proteinuria and preserve renal function in patients with type 1diabetes and moderate renal failure ( 65 ).
The established treatment of diabetes patients with chronic kidney disease has up till now been very similar for type 1 or type 2 diabetes patients. Recent studies have, however, demonstrated that type 2 diabetes patients with impaired renal function have beneficial renal effects beyond the glucose- lowering effects of especially SGLT2i but also GLP-1 RA. Future studies will clarify if new drugs will arrive in the treatment of type 1 diabetes patients with chronic kidney disease.
Declaration of interest
EN has no conflict of interest that could be perceived as prejudicing the impartiality of the research reported. TGJ has received lecture fees from AstraZeneca, Mundipharma, Boehringer Ingelheim, and Novo Nordisk.
This research did not receive any specific grant from any funding agency in the public, commercial or not-for-profit sector.
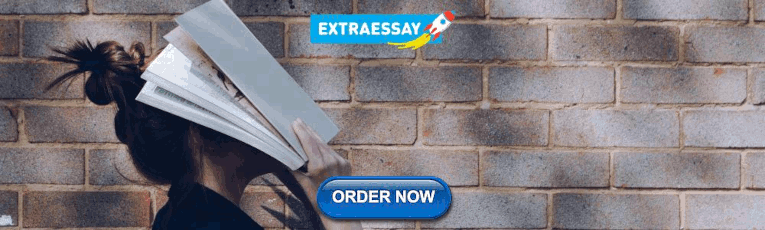
IMAGES
VIDEO
COMMENTS
Location. The positioning of the kidneys is just below the rib cage, with one on each side of the spine. The right kidney is generally slightly lower than the left kidney to make space for the ...
Essay on the Artificial Kidney Essay on the Hormones of the Kidney; Essay # 1. Introduction to Kidney: ADVERTISEMENTS: A large number of waste products are produced in the body as a result of metabolic activities. The main waste products are carbon dioxide, water, and nitrogenous compounds. The retention of these products produces a harmful ...
Kidney. In humans, the kidneys are two reddish-brown bean-shaped blood-filtering organs [1] that are a multilobar, multipapillary form of mammalian kidneys, usually without signs of external lobulation. [2] [3] They are located on the left and right in the retroperitoneal space, and in adult humans are about 12 centimetres ( 41⁄2 inches) in ...
Diseases related to Kidneys. 1. Uremia. In uremia, the kidneys are damaged, and there is a buildup of urea and other toxins in the blood, which is fatal and can cause kidney failure. Patients may experience fatigue, itching, muscle twitching, and loss of mental concentration. The urea can be removed by the process of hemodialysis.
Kidneys are the major organs of the renal system which perform vital homeostatic processes such as maintenance of water and ionic balance in the body. The kidneys' primary function is waste removal through ultrafiltration, leading to urine formation. Moreover, they are actively involved in the reabsorption of amino acids, glucose, and water ...
The regulation of phosphate handling by the kidney, intestine and bone is therefore critical for normal physiology. What is clear from the work presented is that all three of these systems work in an integrated manner and are important for normal phosphate homeostasis. Changes in the levels of any one of these hormones, for example due to ...
The kidneys filter the blood and take out all the waste in the blood. The kidneys send the waste on to the bladder in the form of urine. Kidneys are part of team! Our bodies have important parts that work together just like a team. Some other members of the body's team are the heart, lungs, muscles, brain, and stomach.
Transcript. The kidneys play a vital role in filtering blood and producing urine. Understand how these fist-sized organs receive oxygenated blood, hold onto essential nutrients, and expel waste products. Discover the unique dual capillary system, the vasa recta and peritubular capillaries, that make this process possible.
In addition, about 30% of kidney disease cases are monogenic and could potentially be treated using these genetic medicine approaches. Systemic diseases that involve the kidney, such as diabetes ...
Hypertension is a leading cause of morbidity and mortality and the kidney has a pivotal role in this condition by regulating arterial blood pressure. In this Review, Wadei and Textor provide ...
We included 21 papers of prospective cohort studies with 3-24 years of follow-up. We focused on meat, fish, dairy, vegetables, fruit, coffee, tea, soft drinks, and dietary patterns. There was convincing evidence that a healthy dietary pattern may lower CKD risk. Plant-based foods, coffee, and dairy may be beneficial.
In AKF, symptoms appear suddenly. In CKD, symptoms develop slowly and typically appear after long-term damage has occurred. Symptoms of kidney failure include: swelling of the feet and legs due to ...
Hot Topics in Kidney Health. Join us as we highlight the latest in kidney research, dispel myths, bring you up-to-date news in kidney care, and answer questions from patients to help them live well with kidney disease or a transplant.
Essay On Kidneys. • The process by which an organism obtains its food is called nutrition. • Based on the mode of nutrition plants are classified into autotrophs and heterotrophs. • The plants which can synthesize their own food are called autotrophic plants. • Autotrophs are classified into two types, namely, photoautotrophs and ...
CKD by the numbers. Kidney diseases are a leading cause of death in the United States.; About 35.5 million US adults are estimated to have CKD, and most are undiagnosed.; 40% of people with severely reduced kidney function (not on dialysis) are not aware of having CKD.; Every 24 hours, 360 people begin dialysis treatment for kidney failure. In the United States, diabetes and high blood ...
nephron, functional unit of the kidney, the structure that actually produces urine in the process of removing waste and excess substances from the blood.There are about 1,000,000 nephrons in each human kidney. The most primitive nephrons are found in the kidneys of primitive fish, amphibian larvae, and embryos of more advanced vertebrates.The nephrons found in the kidneys (mesonephros) of ...
essay the anatomy and the histology of the kidneys introduction mr. d is easily thirsty and frequently voids, we suspect there is a problem with is renal. Skip to document. ... Kidneys are retroperitoneal organs, meaning that they lay behind the parietal peritoneum, and anterior to the dorsal body wall. ...
Essays About Kidney. Essay examples. Essay topics. 14 essay samples found. Sort & filter. 1 Kidneys: One of The Most Important Organs . 1 page / 384 words . One of the most important organs in your body is of the kidneys. This is due to the function of the kidneys not only allows your body to detox, here we describe the 5 Remedies to Dissolve ...
CVD and renal consequences. CV abnormalities, even subclinical ones, are frequently associated with a preexistent or de novo chronic kidney disease (CKD): i.e., estimated glomerular filtration rate (eGFR) < 60 ml/min and/or albuminuria or proteinuria, which can progress to end stage renal disease (ESRD) and favor CV morbidity and mortality (so-called chronic CRS).
The kidneys manifest the aging process by steady loss of nephrons and a corresponding decrease in glomerular filtration rate (GFR) beginning about age 30 years. The mechanisms responsible for this observation is are elusive. However, defining chronic kidney disease based on arbitrary, fixed thresholds of GFR in the later phases of life can be ...
Water loss from kidneys: This can result from diuretic use, acute or chronic kidney disease, and hyperglycemia. Water loss from the GI tract: This may result from vomiting , diarrhea , or taking ...
Background Type 2 diabetes mellitus (DM) globally affects 18-20 % of adults over the age of 65 years. Diabetic kidney disease (DKD) is one of the most frequent and dangerous complications of DM2, affecting about one-third of the patients with DM2. In addition to the pancreas, adipocytes, liver, and intestines, the kidneys also play an important role in glycemic control, particularly due to ...
The New England Journal of Medicine has published the most manuscripts on kidney transplantation (n = 26) and is the most cited journal (n = 15,642). The United States has the highest number of publications (n = 61). Kashika is the corresponding author with the most published papers (n = 5; 2892 citations).
Methods. This multicenter, cluster randomized controlled trial evaluated combined endurance and resistance exercise training during hemodialysis versus usual care in chronic kidney failure. It assessed physical functioning, quality of life, hospitalizations, and overall survival. The primary outcome was the change in the 60-second sit-to-stand ...
Kidney Dialysis. Kidney dialysis is used in the event of kidney failure. This is very important as the kidney is the organ in the body which maintains water and ion balance and the level of urea in the body. If left untreated, kidney failure can lead to death. When the body's kidneys only have 10 to 15 percent of their function left people ...
As an important toxic heavy metal, lead exposure can lead to the occurrence of chronic kidney disease (CKD). However, the analysis of its disease burden pattern on a global scale is lacking. This study aimed to analyze the CKD burden attributable to lead exposure globally, regionally and temporally, as well as to examine the role of socio-economic factors. This study used data from the Global ...
Introduction. Diabetes mellitus is among the leading causes of chronic kidney disease and end-stage kidney disease in the western world. It was the most common diagnosis for the initiation of renal replacement therapy in the United States in 2018, accounting for 47% of the cases ().Type 1 and type 2 diabetes mellitus share many clinical characteristics and long-term complications, but they are ...
Biotechnology and Applied Biochemistry publishes original full-length articles, short communications, and reviews of biotechnology. The journal is dedicated to rapid publication of high quality, cutting-edge research at the interface between life sciences and their technological exploitation. The Editors will consider papers for publication based on their novelty, their immediate or future ...