Academia.edu no longer supports Internet Explorer.
To browse Academia.edu and the wider internet faster and more securely, please take a few seconds to upgrade your browser .
Enter the email address you signed up with and we'll email you a reset link.
- We're Hiring!
- Help Center
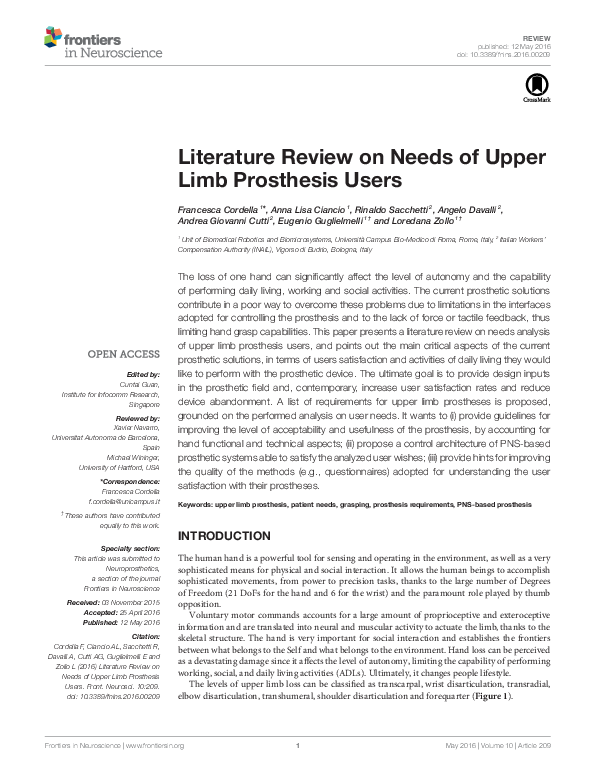
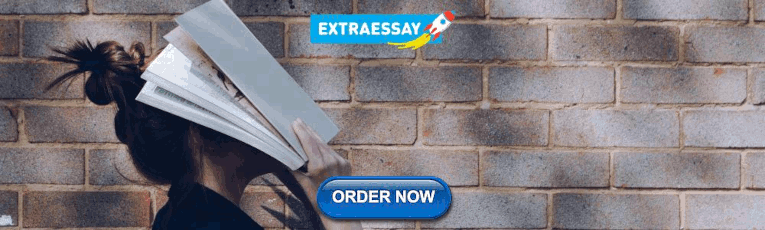
Literature Review on Needs of Upper Limb Prosthesis Users

2016, Frontiers in Neuroscience
Related Papers
Journal of NeuroEngineering and Rehabilitation
Breanne Christie , Cynthia Chestek , Alicia Davis , Susannah Engdahl
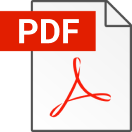
2020 8th IEEE RAS/EMBS International Conference for Biomedical Robotics and Biomechatronics (BioRob)
Numerous assistive devices possess complex ways to operate and interact with the subjects, influencing patients to shed them from their activities of daily living. With the purpose of presenting a better solution to mitigate issues generated by complex or expensive alternatives, a test comparing different user-prosthesis interfaces was elaborated to determine the effects of diverse aspects in their user-friendliness, including that of a version created for this work. A simplistic, anthropomorphic and 3D-printed upper-limb prosthesis was adapted to evaluate all the renditions considered. The chosen design facilitates the modification of its operational mode, facilitating running the tests. Additionally, the selected prosthetic device can easily be adapted to the amputees’ lifestyle in a successful way, as shown by experimental results, providing validity to the study. For the interaction process, a wireless third party device was elected to gather the user intent and, in some renditi...
Expert Review of Medical Devices
Jason Carey
Conference proceedings : ... Annual International Conference of the IEEE Engineering in Medicine and Biology Society. IEEE Engineering in Medicine and Biology Society. Annual Conference
Anindita Saha
The needs of individuals with upper limb amputation and congenital limb difference are not being fully met by current prostheses, as evidenced by prosthesis rejection, non-wear, and user reports of pain and challenging activities. Emerging technologies such as dexterous sensorized robotic limbs, osseointegrated prostheses, implantable EMG electrodes, and electrical stimulation for sensory feedback have the potential to address unmet needs, but pose additional risks. We plan to assess upper limb prosthesis user needs and perspectives on these new benefits and risks using an extensive quantitative survey. In preparation for this survey, we report here on qualitative interviews with seven individuals with upper limb amputation or congenital limb difference. Unstructured text was mined using topic modeling and the results compared with identified themes. A more complete understanding of how novel technologies could address real user concerns will inform implementation of new technologie...
Giorgio Grioli
Sigurður Hólmar Karlsson
Applied Sciences
Lucian Matei
This paper presents aspects of the concept and design of prostheses for the upper limb. The objective of this research is that of prototyping a customized prosthesis, with EMG signals that initiate the motion. The prosthesis’ fingers’ motions (as well as that of its hand and forearm parts) are driven by micro-motors, and assisted by the individualized command and control system. The software and hardware tandem concept of this mechatronic system enables complex motion (in the horizontal and vertical plane) with accurate trajectory and different set rules (gripping pressure, object temperature, acceleration towards the object). One important idea is regarding customization via reverse engineering techniques. Due to this, the dimensions and appearance (geometric characteristics) of the prosthesis would look like the human hand itself. The trajectories and motions of the fingers, thumbs, and joints have been studied by kinematic analysis with the matrix–vector method aided by Matlab. T...
2009 IEEE International Conference on Robotics and Automation
Alicia Davis
Surgically invasive interfaces for upper limb prosthesis control may allow users to operate advanced, multi-articulated devices. Given the potential medical risks of these invasive interfaces, it is important to understand what factors influence an individual's decision to try one. We conducted an anonymous online survey of individuals with upper limb loss. A total of 232 participants provided personal information (such as age, amputation level, etc.) and rated how likely they would be to try noninvasive (myoelectric) and invasive (targeted muscle reinnervation, peripheral nerve interfaces, cortical interfaces) interfaces for prosthesis control. Bivariate relationships between interest in each interface and 16 personal descriptors were examined. Significant variables from the bivariate analyses were then entered into multiple logistic regression models to predict interest in each interface. While many of the bivariate relationships were significant, only a few variables remained...
Julio E. Fajardo
The complexity of the user interfaces and the operating modes present in numerous assistive devices, such as intelligent prostheses, influence patients to shed them from their daily living activities. A methodology to evaluate how diverse aspects impact the workload evoked when using an upper-limb bionic prosthesis for unilateral transradial amputees is proposed and thus able to determine how user-friendly an interface is. The evaluation process consists of adapting the same 3D-printed terminal device to the different user-prosthesis-interface schemes to facilitate running the tests and avoid any possible bias. Moreover, a study comparing the results gathered by both limb-impaired and healthy subjects was carried out to contrast the subjective opinions of both types of volunteers and determines if their reactions have a significant discrepancy, as done in several other studies.
RELATED PAPERS
Stephen Scotti
boshra hatef
CSI Communications, Computer Society of India, New Delhi
VIJAY K U M A R VISHWAKARMA
Dandy Yuliansyah
Science Journal of Clinical Medicine
Rabin bhandari
Bipolar Disorders
DANIELA CAMPANELLA
Dorkis Yamile Cárdenas Casique
Biomacromolecules
Nguyen Tran
World Journal of Neuroscience
David S Younger
New Phytologist
Andrew Groover
Journal of Education Culture and Society
Ligita Stramkale
Respiratory Medicine
Cancer Biology & Therapy
Jurnal Teknik Informatika dan Sistem Informasi
Wildan Mahmud
Aceh Anthropological Journal
Iromi Ilham
PLANT PHYSIOLOGY
william terzaghi
Jurnal CMES
Muhammad Yunus Anis
Mohamed Abdelkader
European Respiratory Review
Jasmin Rostron
Journal of Clinical Medicine
jose Montiel
Gema Wiralodra
Mutiara Salsabiela
Journal of Geophysical Research: Space Physics
David the Brain
Uganda Journal of Agricultural Sciences
Bernard Obaa
Sachin Singh
See More Documents Like This
RELATED TOPICS
- We're Hiring!
- Help Center
- Find new research papers in:
- Health Sciences
- Earth Sciences
- Cognitive Science
- Mathematics
- Computer Science
- Academia ©2024
REVIEW article
Literature review on needs of upper limb prosthesis users.
- 1 Unit of Biomedical Robotics and Biomicrosystems, Università Campus Bio-Medico di Roma, Rome, Italy
- 2 Italian Workers' Compensation Authority (INAIL), Vigorso di Budrio, Bologna, Italy
The loss of one hand can significantly affect the level of autonomy and the capability of performing daily living, working and social activities. The current prosthetic solutions contribute in a poor way to overcome these problems due to limitations in the interfaces adopted for controlling the prosthesis and to the lack of force or tactile feedback, thus limiting hand grasp capabilities. This paper presents a literature review on needs analysis of upper limb prosthesis users, and points out the main critical aspects of the current prosthetic solutions, in terms of users satisfaction and activities of daily living they would like to perform with the prosthetic device. The ultimate goal is to provide design inputs in the prosthetic field and, contemporary, increase user satisfaction rates and reduce device abandonment. A list of requirements for upper limb prostheses is proposed, grounded on the performed analysis on user needs. It wants to (i) provide guidelines for improving the level of acceptability and usefulness of the prosthesis, by accounting for hand functional and technical aspects; (ii) propose a control architecture of PNS-based prosthetic systems able to satisfy the analyzed user wishes; (iii) provide hints for improving the quality of the methods (e.g., questionnaires) adopted for understanding the user satisfaction with their prostheses.
Introduction
The human hand is a powerful tool for sensing and operating in the environment, as well as a very sophisticated means for physical and social interaction. It allows the human beings to accomplish sophisticated movements, from power to precision tasks, thanks to the large number of Degrees of Freedom (21 DoFs for the hand and 6 for the wrist) and the paramount role played by thumb opposition.
Voluntary motor commands accounts for a large amount of proprioceptive and exteroceptive information and are translated into neural and muscular activity to actuate the limb, thanks to the skeletal structure. The hand is very important for social interaction and establishes the frontiers between what belongs to the Self and what belongs to the environment. Hand loss can be perceived as a devastating damage since it affects the level of autonomy, limiting the capability of performing working, social, and daily living activities (ADLs). Ultimately, it changes people lifestyle.
The levels of upper limb loss can be classified as transcarpal, wrist disarticulation, transradial, elbow disarticulation, transhumeral, shoulder disarticulation and forequarter (Figure 1 ).
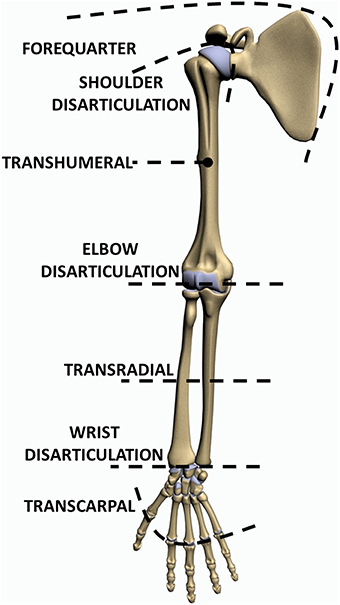
Figure 1. Levels of upper limb absence .
Around 541,000 Americans suffered from different levels of upper limb loss in 2005; the number of cases is expected to double at least by 2050 ( Ziegler-Graham et al., 2008 ). Approximately 3500 and 5200 upper limb amputations are reported each year in Italy 1 and in UK, respectively. The incidence of the different levels of upper limb loss is also shown in Figure 2 : 16% trans-humeral, 12% transradial, 2% forequarter, 3% shoulder disarticulation, 1% elbow disarticulation, 2% wrist disarticulation, 61% transcarpal, and 3% bilateral limb loss. Traumatism is the first cause of upper limb amputation, predominantly for males 2 . It is followed by neoplasia and vascular or infectious diseases ( Frontera and Silver, 2014 ).
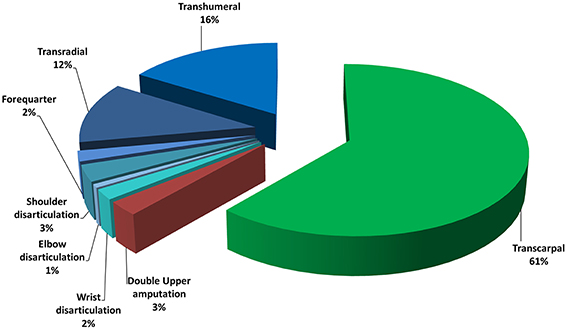
Figure 2. Statistics on level of upper limb absence in Italy and United Kingdom .
The loss of a limb interrupts the closed-loop with the brain that takes place by means of the efferent and afferent pathways, responsible for motor control and sensory feedback, respectively.
Upper limb prostheses ( Fumero and Costantino, 2001 ) can be classified into two main categories on the basis of their functioning: passive prostheses (which in turn are divided into cosmetic and functional) and active prostheses (which include body-powered and externally powered).
Cosmetic prostheses mainly aim at the aesthetic substitution of the missing body part, while functional prostheses have the purpose of facilitating very specific activities, such as those related to work or sport.
Within the active prostheses, the body-powered ones are controlled by cables fastened to the sound limb of the amputee by means of harnesses. The high expenditure of energy required to the user is one of the drawbacks of this kind of prostheses. Instead, externally powered prostheses exploit an external power source to raise the energy needed for movement, for example a battery pack. They can be further classified into two sub-categories: myoelectric (which are controlled through electromyographic (EMG) signals) and electric (ideal for example for phocomelic people who can command the prosthesis by means of external buttons).
Despite the advances in technology over the last 50 years, today's upper limb prostheses are still affected by relevant limitations. One of the main engineering challenges in the development of prosthetic devices is, on one hand, to embed actuators, sensors and electronic components into a prosthesis of the same size and weight of the replaced hand or limb, on the other hand, to improve prosthesis control that notably affects functionality.
Intuitive control can be developed by extracting the user's intention from signals recorded in a non-invasive way [through surface EMG electrodes (sEMG; Dohnalek et al., 2013 ), ultrasound imaging ( Gonzales and Castellini, 2013 ), force myography (FMG; Wininger et al., 2008 )] or else in an invasive way [by means of Implantable Myoelectric Sensors (IMES; Pasquina et al., 2015 ), neural interfaces ( Dhillon et al., 2004 )] from the Peripheral or Central Nervous System. Invasive surgical procedures, such as Targeted Muscle Reinnervation (TMR), have been applied in some cases of very proximal limb loss to allow an intuitive prosthesis control through myoelectric interfaces.
Several researches have been carried out in this field, but a lot of effort is still required for improving the interfaces. Desired requirements are: (i) real-time, direct, robust and simultaneous control of multiple DoFs in a natural and intuitive manner, (ii) bidirectional communication with the Peripheral Nervous System (PNS), and (iii) fast learning. In most of the existing control strategies, limited information (i.e., shoulder movements or recorded EMG signals) is used for activating several DoFs of the artificial limb, thus making non-intuitive and unnatural prosthesis control and requiring a huge cognitive load. Classical myoelectric control is based on on/off ( Scott and Parker, 1988 ) or proportional techniques ( Fougner et al., 2012 ). Notwithstanding their wide adoption in the commercially available systems as well as in the clinical practice ( Jiang and Farina, 2014 ), they do not allow the user to simultaneously control more than one DoF (far from the multifunctional control of the natural hand). Moreover, they require a long training phase and suffer from signal degradation due to sweating or inadequate positioning of the socket.
In order to overcome these limitations, several approaches have been proposed, such as ultrasound imaging ( Gonzales and Castellini, 2013 ), FMG ( Wininger et al., 2008 ), TMR ( Hijjawi et al., 2006 ; Miller et al., 2008 ), pattern recognition techniques ( Cloutier and Yang, 2013 ) applied to EMG signals acquired through implantable (IMES; Pasquina et al., 2015 ) or surface electrodes ( Dohnalek et al., 2013 ), and neural signals acquired through implantable neural interfaces ( Dhillon et al., 2004 ; Polasek et al., 2009 ; Rossini et al., 2010 ).
Most of the aforementioned solutions are still developed in the research field (e.g., ultrasound imaging, neural interfaces, IMES, pattern recognition) without clinical application. Pattern recognition offers the advantage of enabling the simultaneous, independent control of multiple DoFs ( Ortiz-Catalan et al., 2014 ). Nonetheless, performance is affected by arm posture modification, electrode positioning, fatigue, inherent cross talk in the surface signal and displacement of the muscles during contraction. Pattern recognition also requires very long learning sessions; further, its performance in the real context is different from the laboratory settings ( Jiang and Farina, 2014 ), thus limiting its clinical applicability and acceptance. In January 2015 the first device based on pattern recognition and surface electrodes (COAPT 3 ) has been commercialized.
The adoption of IMES has been tested on transradial amputees ( Pasquina et al., 2015 ) to provide intuitive and stable myoelectric control. Promising preliminary results have been obtained (i.e., the prosthesis control is more robust to limb position and environmental conditions with respect to the use of superficial electrodes), but IMES cannot be employed when the sensing sites are very close each other, or else if the target muscle is small or thin.
Ultrasound imaging has a comparable accuracy with sEMG, but it has lower wearability and is much more sensitive to arm/hand displacement. Further efforts could make this solution a valuable alternative to sEMG ( Castellini et al., 2014 ), especially working on signal processing, component miniaturization, force decoding ( Gonzales and Castellini, 2013 ).
Force myography ( Wininger et al., 2008 ) consists in placing force sensing resistors (FSRs) on the limb surface and detecting the volumetric changes of the residuum. It can provide information about the user's motion intention ( Li et al., 2012 ) and grip force ( Wininger et al., 2008 ), and does not depend on a precise placement of the sensors. However, preliminary results show that grasp classification accuracy is still lower than EMG ( Cho et al., 2016 ).
Natural control occurring through the PNS can be achieved by means of peripheral neural interfaces ( Navarro et al., 2005 ). The main limitations of intraneural electrodes are related to the high invasiveness and signal degeneration due to fibrotic reaction; additionally, the computational burden for signal processing and classification on the efferent pathway is considerably higher than for EMG ( Cloutier and Yang, 2013 ). On the other hand, it is really effective in returning tactile perception on the afferent pathway ( Raspopovic et al., 2014 ).
As for neural interfaces, invasiveness is a drawback also for TMR and IMES. In Engdahl et al. (2015) it was shown that the surgical risk is the main concern of individuals with upper limb loss about using invasive techniques for prosthesis control. An online questionnaire proposed to 104 American people outlined that, notwithstanding the preference for non-invasive interfaces (83% was interested in EMG control), invasive techniques can be accepted when a higher level of functionality is offered (63% expressed interest in TMR and 68% in neural interfaces).
While control of upper limb prostheses is still coarse, progress in mechanics ( Atzori and Muller, 2015 ) is notable, as confirmed by the advanced poliarticulated myoelectric prosthetic hands available on the market (i.e., the i-Limb 4 , the Bebionic 5 , and the Michelangelo 6 hands in Figure 3 ; Belter et al., 2013 ). They enable several grasping tasks thanks to the number of DoFs, as shown in Table 1 .
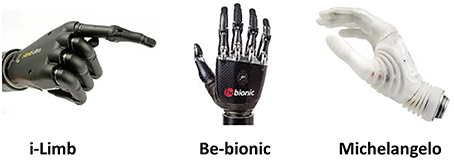
Figure 3. Most advanced commercially available prosthetic hands .
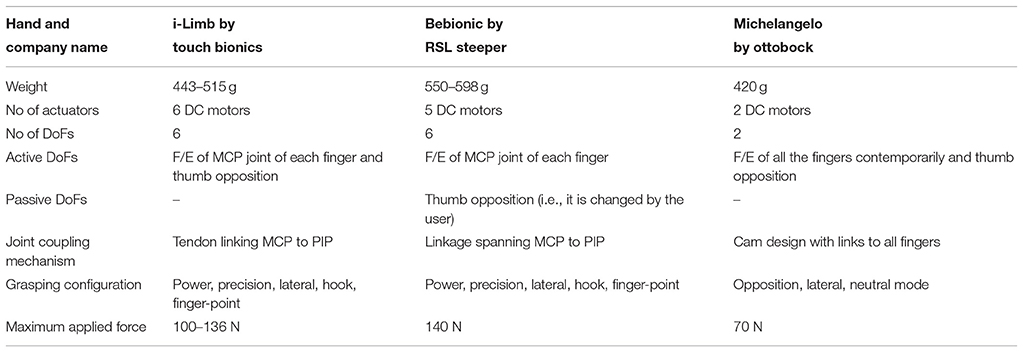
Table 1. Characteristics of poliarticulated commercially available prosthetic hands ( Belter et al., 2013 ) .
One of the main limitations of current devices is represented by the lack of an intuitive and reliable interface able to map the user motion volition to real movement of the prosthesis ( Dhillon and Horch, 2005 ). Finally, the necessity of an extensive training required to properly manage the artificial hand, the lack of sensory feedback and the noise produced by the actuators during movements make the prosthetic hands still far from fully addressing the users' needs ( Clement et al., 2011 ), see Section Needs Analysis of Upper Limb Prosthesis Users. Therefore, this paper intends to perform an updated literature review on the analysis of user needs; the ultimate goal is to provide valid indications on the requirements that prostheses should have to increase user acceptability. In Section Aim of the Study, the study purposes will be explained in detail.
The paper is structured as follows: Section Aim of the Study presents the aim of this study; Section Method describes the method followed for performing this review; Section Needs Analysis of Upper Limb Prosthesis Users provides an overview of the studies on user needs, while Section Discussion reports the requirements to be satisfied by the prosthetic system in order to meet the patient's needs. Finally, Section 6 offers critical considerations and suggests future pathways of development.
Aim of the Study
This paper intends to carry out an in-depth study of the literature on needs analysis of upper limb prosthesis users in order to consolidate current knowledge in the field and delineate the users' priorities as well as main requirements for upper limb prostheses. Although individual solutions (in terms of mechatronic design, socket and control) could be proposed for each single user in order to meet the patient's specific needs, general insights can be provided for improving user satisfaction, thus paving the way to subsequent user-tailored solutions.
This work has the twofold purpose of (i) helping focus research efforts toward the development of prostheses that are functional and appealing for individuals with upper limb loss, by addressing their needs; (ii) providing foundations for future studies to more in-depth explore user needs and priorities that inevitably can change with technology advancements and market development.
The expected added value provided by this work is to complete the current knowledge on the users' needs with more recent papers, by critically evaluating and comparing (when possible) the available results, and pointing out inconsistencies and neglected aspects. Hence, an updated list of user requirements and prosthesis features has been built and is available for the research community. The ultimate goal is to provide indications for the development of future prosthetic technologies that are appealing to individuals with upper limb loss.
A wide literature search updated to March 2016 has been performed from the following databases: PubMed, Google Scholar, Cochrane Database of Systematic Reviews. The keywords (and their combinations) adopted for the research are the following: upper limb, prosthesis, amputation level, upper limb loss, myoelectric, body-powered, cosmetic, prosthesis control, user needs, satisfaction, priorities, abandonment, sensory feedback. All publications in English and Italian languages appeared between 1980 and 2016 have been considered. Additional articles extracted from the references of the papers identified in the electronic search have been included. The inclusion criteria for selecting the publications relevant for the review purpose are the following: studies on upper limb prosthesis users that account for cosmetics, functionality and comfort; studies on upper limb prosthesis users who wear cosmetic, body-powered and myoelectric prostheses; studies reporting user opinions about desired features, design priorities and activities of daily living they desire to perform with the prosthesis.
A flowchart of the search and inclusion process is shown in Figure 4 . A total of 354 papers has been gathered by using the aforementioned search method. The abstracts matching the inclusion criteria have been selected. When appropriate, the full paper has been read. Therefore, from the initial 354 papers, 331 have been excluded since they did not meet the inclusion criteria. The remaining 23 papers have been carefully read. Sixteen of these have been excluded from the following discussion since already reviewed several times in the literature or because the reported data were not significant for the purposes of this work. The last seven studies will be discussed in the following. They also include the results of previous studies that are not explicitly mentioned in this review.
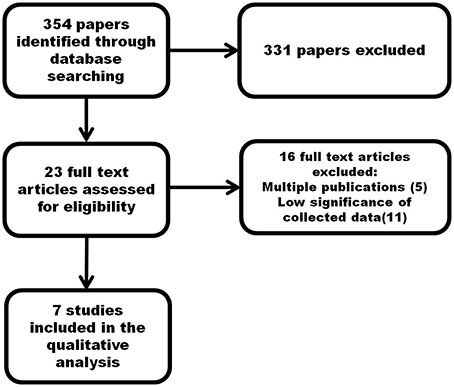
Figure 4. Flowchart of the search and inclusion process .
The selected studies adopted different methods and tools, and provide heterogeneous data that are difficult to be analyzed in a systematic way. However, despite the difficulty to find a primary outcome measure, interesting common features have been extracted and a list of factors necessary to assess user demands on prostheses has been identified.
Needs Analysis of Upper Limb Prosthesis Users
The main issues faced by the literature over the years are related to: the type of activities that the prostheses can help perform ( Van Lunteren et al., 1983 ) or that could be performed with a prosthesis ( Fraser, 1998 ); the explanation of why several amputees prefer not to use the prosthesis ( Fraser, 1993 ; Kejlaa, 1993 ; Biddiss and Chau, 2007 ; Peerdeman et al., 2011 ); the identification of the problems related to job before and after amputation ( Heger et al., 1985 ; Wright et al., 1995 ). However, the studies often use different methods of analysis and adopt different selection criteria of the involved users; moreover, they are also limited to a single site or country. In the attempt of performing a comprehensive analysis of the users' needs, the selected papers were in-depth analyzed and differences and similarities were reported, aiming at identifying general requirements for several users.
The seven selected relevant studies are Kyberd and Hill (2007) , Biddiss et al. (2007) , Jang et al. (2011) , Pylatiuk et al. (2007) , Østlie et al. (2012) , Bouffard et al. (2012) , and Lucchetti et al. (2015) . Special attention has been dedicated to the comparative analysis of user experience with myoelectric, electric, body-powered and passive upper limb prostheses, independently of the level of limb loss. In all the studies, questionnaires were used to collect data from upper limb prosthesis users. In Lucchetti et al. (2015) , a questionnaire was used for physiological assessment, and practical tests were adopted for assessing functional abilities. In Østlie et al. (2012) , questionnaires, clinical tests and interviews were used. Three studies, i.e., Biddiss et al. (2007) , Jang et al. (2011) , and Pylatiuk et al. (2007) , involved both adults and children, while the others Kyberd and Hill (2007) , Østlie et al. (2012) , Bouffard et al. (2012) , and Lucchetti et al. (2015) were focused only on adults.
The number of subjects involved in the experimental groups ranged from 6 in Lucchetti et al. (2015) to 307 in Jang et al. (2011) (for a total of n = 958 users). All the seven analyzed studies are focused on the analysis of myoelectric prosthetic users, while four of them report priorities and satisfaction levels of body-powered prosthesis users ( Biddiss et al., 2007 ; Jang et al., 2011 ; Østlie et al., 2012 ; Bouffard et al., 2012 ), and four studies are concerned with cosmetic prosthesis users ( Kyberd and Hill, 2007 ; Biddiss et al., 2007 ; Jang et al., 2011 ; Østlie et al., 2012 ).
The participants were asked to answer questionnaires about functional, aesthetical and commercial aspects of the prostheses.
The studies are structured as follows and details are reported in Table 2 :
• In Kyberd and Hill (2007) a study performed in Sweden and UK on 156 patients with unilateral upper limb prostheses is reported. The analysis involved users with different levels of limb loss using myoelectric and cosmetic prostheses. A low percentage of users wore other types of prostheses, but the authors did not provide data regarding their answers.
• In Biddiss et al. (2007) , 242 users with different levels of limb loss were asked to provide a list of priorities of prosthetic hand features, sorted by relevance. The authors did not account for the type of limb loss in the data analysis. However, it was observed that individuals with above elbow or bilateral amputation preferred body-powered hooks, while adults with congenital limb loss chose cosmetic hands.
• In Jang et al. (2011) the results from 307 Korean subjects were reported, including 34 bilateral amputees. The analysis involved users with different levels of amputation mainly using cosmetic devices.
• In Pylatiuk et al. (2007) , the satisfaction of myoelectric hand users with different levels of amputation has been evaluated by means of an internet survey. 54 German users (34 males, 9 females and 11 children) answered questions about (i) general information regarding the amputation (such as date, reason, level, used prosthesis); (ii) time of use of the prosthesis; (iii) perceived noise, weight and appearance of the prosthesis; (iv) activities where the prosthesis is used and requirements for future improvements.
• In Østlie et al. (2012) , the perceived usefulness of the prosthesis and the skills of prosthesis users in ADL tasks were evaluated by means of questionnaires, clinical tests and interviews. Two forty four Norway users compiled questionnaires with the objective of identifying the “pattern of prosthesis wear” (i.e., type and wearing times of prosthetic devices) and assessing the prosthesis usefulness. Fifty users were involved in clinical tests and interviews on 59 tasks, with the aim to evaluate user skills while performing ADLs.
• In Bouffard et al. (2012) , 12 people from Quebec with an amputation proximal to the wrist were interviewed. The main purpose of the work was to investigate the influence of the phantom limb pain on the prosthesis use. The satisfaction of the users with their prosthesis was also analyzed.
• In Lucchetti et al. (2015) , the results of practical tests and questionnaires on the functional and psychosocial impact of a transradial myoelectric prosthesis was reported. The study involved 6 Italian subjects who compared experience and performance achieved with two different prosthetic hands (i.e., the Michelangelo hand and a traditional tridigital myoelectric prosthetic hand by Otto Bock©).
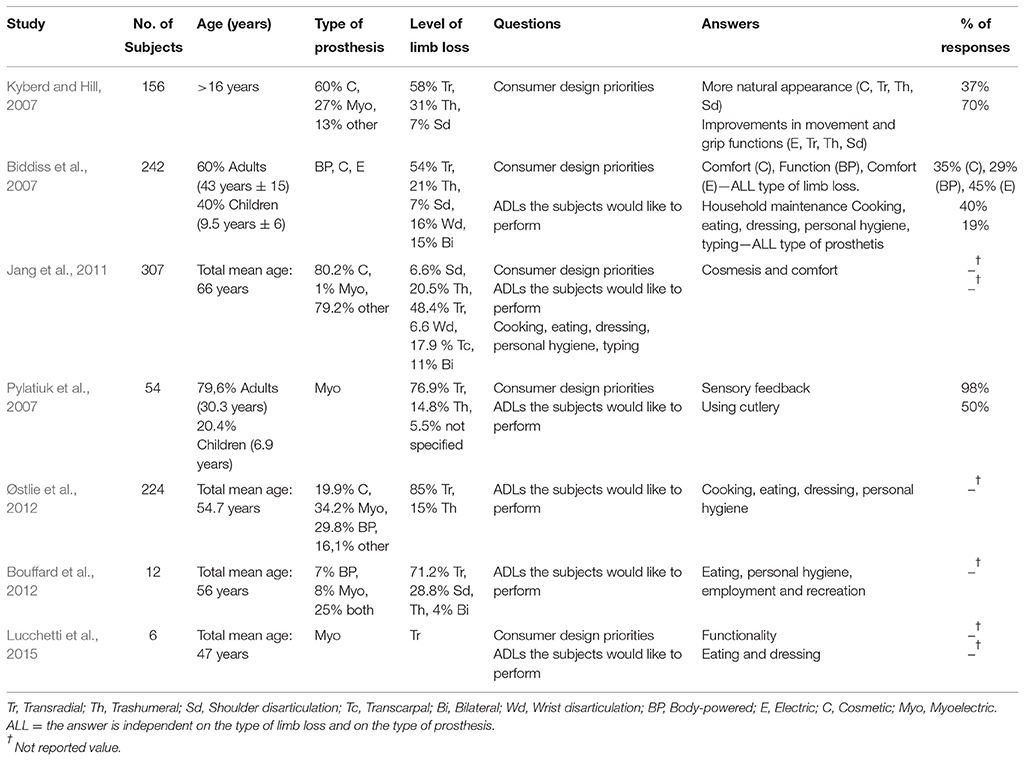
Table 2. Summary of the reported analysis .
Before going in-depth in the results of the aforementioned studies, it is worth observing that the evaluation of usefulness and patient satisfaction for different types of prostheses is notably influenced by the unilateral or else bilateral loss ( Davalli and Sacchetti, 2009 , in Italian). In case of unilateral patients, the prosthetic device is mostly an aid for the sound limbs, while in case of subjects with bilateral limb loss the prosthesis is the main way to interact with the environment.
This aspect has not adequately been faced in the analyzed studies. In fact, the percentage of the respondents with a bilateral limb loss was typically low: 15% in Biddiss et al. (2007) , 11% in Jang et al. (2011) , 4% in Østlie et al. (2012) , for a total of 77 bilateral amputees. In Kyberd and Hill (2007) , Bouffard et al. (2012) , and Lucchetti et al. (2015) only unilateral upper limb prosthesis users were considered, while in Pylatiuk et al. (2007) the side of limb loss is not specified. The low percentage with respect to the total number of participants led the authors to report only global results, without distinguishing between unilateral and bilateral prosthetic users. Only in Jang et al. (2011) considerations about prosthesis users with bilateral upper limb loss have been presented. They regard the level of satisfaction, the wearing time, the most difficult ADLs and the occupational status. Over 34 bilateral amputees, 26.5% were “dissatisfied,” 38.2% were “moderately satisfied” and 35.3% were “satisfied” with the currently used prostheses. The usage time of the prosthesis was 8–16 h a day for 67.6% of subjects, 4–8 h for 11.8%, and less than 4 h for 20.6%. The most difficult ADLs resulted to be: tying shoe laces (82.4%), buttoning shirts (79.4%) using scissors (76.5%), opening and drinking a bottle beverage (67.6%) and washing face (58.8%). None of the bilateral prosthesis users continued the same work after the amputation: 14.7% were employed on different tasks, while 85.3% did not return to work.
The study in the literature involving the highest number (i.e., 117) of subjects with bilateral upper limb loss is ( Atkins et al., 1996 ). The results of this study have not been explicitly analyzed in this paper, being an old study already accounted for in the more recent papers discussed here. Nevertheless, it is worth reporting the interesting result that users with bilateral amputation aspired to handle efficiently a large variety of objects, apply high forces and finely control motion thanks to the presence of force sensory feedback ( Heckathorne, 1994 ; Atkins et al., 1996 ).
Two topics faced in the selected studies are the cost and the maintenance of the prosthesis. Users with body-powered prostheses believe that the cost of their prosthesis is adequate and that the cost for glove replacement is a primary concern; on the other hand, users of myoelectrical prosthesis consider too high the cost of the device and indicate the replacement of gloves and battery as the main maintenance issues. This is also supported by Blough et al. (2010) , where the cost analysis on 5-year show that emerging high technology devices will inevitably lead to increasing costs. The cost factors are also related to the different reimbursements policies applied by insurance companies and public healthcare systems.
A comparative analysis of these studies has pointed out three important issues for users wearing body-powered prostheses: (i) the important role of wrist movements; (ii) the need of reducing the visual attention during task execution; (iii) the possibility of coordinating joint movements.
Conversely, users wearing myoelectric prostheses wished to reduce the visual attention and have flexing fingers, thumb opposition to each finger.
The most frequently asked questions are related to (i) general information regarding the limb loss (such as date, reason, level, used prosthesis); (ii) period of time during which the prosthesis is worn during the day; (iii) degree of satisfaction of the prosthesis characteristics, such as aesthetics, weight, comfort, sweating, wrist movements; (iv) level of satisfaction of the prosthesis functional capabilities; (v) activities that the users desire to perform with their prosthesis.
In particular, in Kyberd and Hill (2007) it has been shown that many participants wore the prosthesis at least 8 h a day and used it mainly in activities such as working, driving and sports. Similar results have been shown in Pylatiuk et al. (2007) and Østlie et al. (2012) , where the users declared to use the prosthesis for at least 8 h a day especially during work, and in Bouffard et al. (2012) , where 75% of the users wore their prosthesis for more than 6 h a day. On the other hand, in Jang et al. (2011) less than half of the amputees (44.7%) used their cosmetic prostheses for at least 8 h a day.
With respect to the degree of satisfaction of the prosthesis characteristics, the importance of different design characteristics for users with different prostheses (passive, body-powered, myoelectric) and the importance of the functional role for active and passive prostheses are reported in Biddiss et al. (2007) (Tables 3 , 4 , respectively). The ranking of importance is obtained on the basis of the Friedman's Rank Test and multiple answers were proposed in the questionnaire.
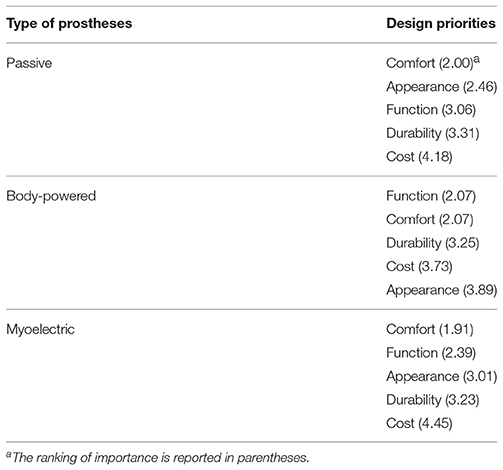
Table 3. Design priorities of passive, body-powered and myoelectric prosthesis ( Biddiss et al., 2007 ) .
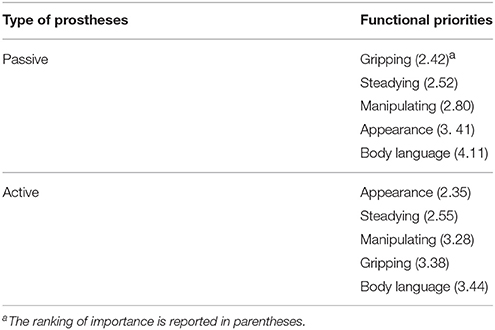
Table 4. Consumer specified importance of functional roles for passive and active prostheses ( Biddiss et al., 2007 ) .
In Biddiss et al. (2007) design priorities for future improvements for children and adults were concerned with weight, overall appearance and comfort (Table 5 ). This was also confirmed by Pylatiuk et al. (2007) , where 77% of respondents judged the prosthesis too heavy. No information about the level of limb loss was reported. In Pylatiuk et al. (2007) as well as in Østlie et al. (2012) the cosmetic appearance obtained the higher degree of satisfaction (50 and 82.2%, respectively).
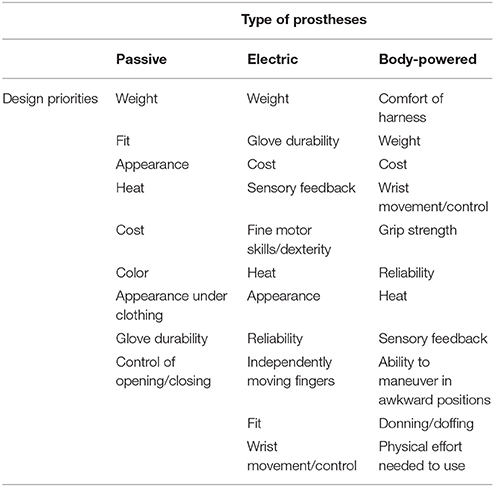
Table 5. Consumer design priorities emerged from the open-ended question ( Biddiss et al., 2007 ) .
The prosthesis functional capabilities were analyzed in Kyberd and Hill (2007) , where a number of limitations were reported by the subjects notwithstanding the high level of user satisfaction [mean value 6.8 in the range 1 (very dissatisfied) to 10 (very satisfied)]. They mainly regard the impossibility of the prosthesis to adapt the grasping configuration to the object shape, the need for higher precision (as also reported in Biddiss et al., 2007 ), and the difficulty to wear gloves. Table 6 reports the features identified by the users as the most important to be improved. Often, the same improvements are required for electric and cosmetic prostheses, although with different level of importance. For instance, the features related to movement and grip functions have greater importance for myoelectric prosthesis users rather than for cosmetic prosthesis users. In Pylatiuk et al. (2007) the noise produced by the prosthesis (evaluated too high by 25% of respondents) and the prosthesis grasping speed (considered too slow by 80% of respondents) are two aspects to be improved. These complaints are confirmed in Jang et al. (2011) , where only 30% of respondents reported satisfaction with the prosthesis.
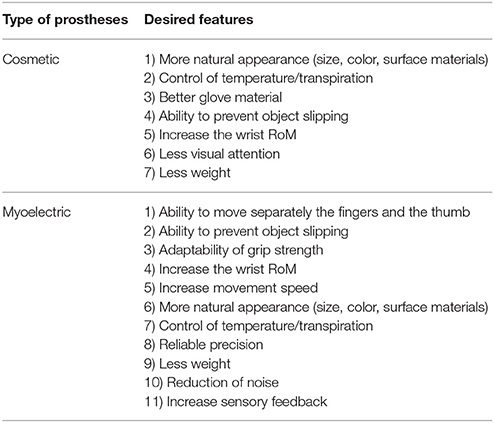
Table 6. Prioritization of the features to improve for prosthetic users ( Kyberd and Hill, 2007 ) .
In the selected studies, many challenging or desired activities have been identified. In particular, in Biddiss et al. (2007) , these activities are related to household maintenance, heavy lifting, sports, activities of daily living (i.e., cooking, eating, dressing, personal hygiene, typing) and hobbies (i.e., playing a musical instrument). The most desired activity is using cutlery, followed by handicrafts, personal hygiene, opening and closing a door, dressing and undressing; the least wanted activity is writing with the prosthesis. Furthermore, most users (98%) desire to feel the force applied by the prosthesis during grasping, followed by feeling the temperature and performing additional movements, such as pointing the index finger, moving individual fingers, performing wrist flexion/extension, decreasing visual attention and improving cosmetic glove material. These results are confirmed by Jang et al. (2011) and Lucchetti et al. (2015) . In fact, the analysis has pointed out that user priorities in terms of ADLs and grasps are eating, dressing and personal hygiene. Additional fundamental features requested by the users are functionality, cosmetics, improvement of the gloves, wrist motion, reliability, noise, dimension and weight.
The answers allowed us to extract from each reviewed study the consumer design priorities and the ADLs the subjects would like to perform (listed in Table 2 ).
In Table 2 type of prosthesis, level of absence, population, answered questions and percentage of response have been reported for each study. The percentages of unilateral and bilateral limb loss, of people wearing the different types of prosthesis and of different levels of limb loss are also listed in the table, when available.
The table allows the extraction of some general considerations on the users' needs, which are summarized in the following.
• As shown in Kyberd and Hill (2007) , it is worth observing that similar interests and needs have been reported by European and American participants.
• When considered, the level of limb loss did not produce significant differences in the desired features or activities to be performed. However, it is worth remarking that the analyzed studies do not investigate the effect on the expressed needs of the type of limb loss (i.e., unilateral vs. bilateral), which is expected to produce significant differences, as reported in Section Discussion.
• The low level of satisfaction for comfort, appearance and functionality is quite common among the users, in particular for cosmetic and body-powered prostheses. This is confirmed by the percentage of prosthesis use abandonment (20–30%), especially due to the lack of functionality, comfort, appearance and sensory feedback ( Biddiss and Chau, 2007 ). The satisfaction level as well as the prosthesis choice could also depend on cultural, social and working factors. In fact, body-powered prostheses, being characterized by moderate cost, durability and reliability, are preferred by workers who are in labor-intensive manual and outdoor occupations. They do not frequently use myoelectric prostheses (preferred by office workers) since, compared to body-powered prostheses, they are more susceptible to be damaged during hard work, present slower response time and require a long lasting learning phase.
• As reported in Biddiss et al. (2007) , body-powered prostheses are preferred in USA, while in Europe cosmetic and myoelectric prostheses are mostly adopted. It could also depend on public health care resources, different in each country.
• According to Kyberd and Hill (2007) and Biddiss et al. (2007) it is possible to conclude that user priorities vary depending on the type of prosthesis. For instance, cosmetic users priorities are prosthesis appearance and comfort; on the other hand, myoelectric and body-powered users pay more attention to function and comfort. Improvements in movement and grip functions are primary requirements for myoelectric prosthesis users. Several studies pointed out the wish to control the grip strength and avoid object slippage.
• Myoelectric prostheses are the most efficient in terms of dexterity and interface intuitiveness (compared to cosmetic and body-powered ones), thanks to the higher functionality and the reduced expenditure of energy required to the user. However, myoelectric users complain about the lack of sensory feedback, which is indicated as the most desired design priority (ranked with the highest score in Pylatiuk et al., 2007 ).
The analyzed studies lack of a common evaluation criteria of the user satisfaction regarding prosthetic usefulness. In fact, in Kyberd and Hill (2007) satisfaction has been rated on a 5-point scale and in a 10-point scale, respectively, ranging from “very satisfied” (5 or 10) to “very dissatisfied” (1); in Jang et al. (2011) the evaluation scale consisted of subjective judgments (i.e., very satisfied, quite satisfied and very unsatisfied), in Biddiss et al. (2007) , Pylatiuk et al. (2007) , Østlie et al. (2012) , and Jang et al. (2011) , the Likert scale and a priority score value have been adopted; the study in Bouffard et al. (2012) adopts the rating used in the questionnaire for Quebec user evaluation of satisfaction with assistive technology. This absence of uniformity makes difficult to extract objective data, allows us to infer only qualitative information. The introduction of practical tests (i.e., SouthHampton Hand Assessment Procedure, Box and Block, etc.), as in Lucchetti et al. (2015) , could be useful to perform an objective functional assessment. Analogously in Lindner et al. (2010) measures providing reliable information on the device functionality have been identified.
The obtained data certainly stress the aspect that future research and developments should be consumer-driven.
The performed analysis shows that there is a significant variability in the population of upper limb prosthesis users, due to individual anatomy, lifestyle, etc. Therefore, individual solutions should always be considered for the type of prosthesis, the socket, the control and the training. Nevertheless, needs and requirements in common among the users can be found. In particular, the analysis of the literature on the user needs and priorities allows the definition of the following list of user requirements:
• Performing activities of daily living mainly related to eating and dressing, such as combining fork and knife motion, cutting the meat, handling buttons, tying shoelaces. This is desired by all the users, independently either of the level of limb loss (e.g., transradial, transhumeral) or the type of prosthesis (e.g., body-powered, myoelectric). Therefore, a prosthetic system is required to perform basic grasping actions (corresponding to power, pinch, lateral, neutral and pointing of the index finger) and simple manipulation tasks enabling the execution of ADLs.
• Providing the user with sensory feedback. This requirement is shared by all the users, independently of the type of prostheses and level of limb loss (Tables 3 , 6 ).
• Reliability of hand battery and electrodes for active prostheses users (Tables 5 , 6 ).
• Ability to perform activities with higher strength. The priority for myoelectric and body-powered prosthesis users of controlling the grasping force and applying high level forces is reported in Tables 5 , 6 .
• Performing actions in a more coordinated manner and with less visual attention. These are skills desired by all the users (Table 6 ).
• Need to wear prostheses with a high level of anthropomorphism (in terms of size, weight, shape, and color). It is a priority for cosmetic prosthesis users; however, also active prosthesis users are interested in anthropomorphism, as shown in Tables 3 , 5 , 6 .
• Performing stable grasps, thus avoiding slippage. As evident from Tables 4 – 6 , this is desired by all the users. The use of slip sensors and embedded control might be used to face this issue.
• Changing position and orientation of the grasped object, thus entailing capabilities of object manipulation (Tables 3 , 5 ). This is a priority for active prosthesis users.
• Ability to move each finger independently, as in free manipulation. As evident in Tables 5 , 6 , this is required by myoelectric prosthesis users.
• Improving the performance of thumb, index and middle finger (Table 6 ).
• Improving precision and efficient handling of small objects. The study in Biddiss et al. (2007) pointed out the need for improved dexterity and fine motor skills.
• Improvement of heat dissipation (Tables 5 , 6 ). This is wished by all the users.
• Reduction of the active prosthesis motor noise (Table 6 ).
• Prosthetic wrist with at least one degree of passive flexion/extension, given the fundamental role of the wrist in ADLs (Tables 5 , 6 ).
• Improving the variety of gloves (flexible, resistant, hardly getting dirty), making them compatible with very common activities, such as the use of touch screens ( Lucchetti et al., 2015 ).
• Providing the prosthesis with an open hand configuration close to the natural one ( Lucchetti et al., 2015 ).
• Improving device duration (estimated around 150,000 cycle/year; Lucchetti et al., 2015 ).
It is interesting to note that some of the requirements emerging from the analyzed studies were already present in a 20-years-old paper ( Atkins et al., 1996 ). This represents one of the largest investigations about upper limb amputees (involving 1575 subjects) and a fundamental study on priorities of users wearing body-powered and myoelectric prostheses. Several issues in the Atkins's paper are still supported by recent papers, such as the feeling about the prosthesis costs and the demand for reducing visual attention, increasing the wrist RoM and independently moving the fingers.
Additionally, the performed literature review has allowed us to identify the functional tasks required by the prosthesis users. They are basic movements, e.g.,:
• grasping configurations: lateral, pinch, hook, spherical, cylindrical grasp, flattened hand and the centralized grip
• neutral position: complete hand opening, with a natural configuration
• manipulation movements: rotation, slipping, translation, pointing index and pushing coin,
that can be combined to generate the complex ADLs that the patient desire to perform with the prostheses, such as eating (i.e., using knife and fork, cutting the meat, drinking from a glass), dressing (i.e., tie shoelaces, handling of clothes), type writing, handling cell phone, and opening a door ( Biddiss et al., 2007 ; Kyberd and Hill, 2007 ; Cloutier and Yang, 2013 ).
The possibility of executing the grasping and manipulation operations listed above also depends on the kinematic and mechanical features of the poliarticulated prostheses. To this purpose, some basic requirements for the hands can be inferred from the user needs analysis: at least two DoFs for the thumb (the former for opposition, which may also be passive, and the latter for flexion/extension), one DoF for index flexion/extension and one DoF for flexion/extension of the remaining fingers, in the hypothesis of finger underactuated mechanisms. Although such requirements and configurations can be satisfied by most of the commercially available poliarticulated prosthetic hands (as shown in Section Introduction) and hand prototypes, a number of limitations still remain concerning the level of dexterity and grasp capability. This is mainly due to control strategies that are not able to manage and properly combine basic hand movements to generate the desired complex motion.
In order to improve the prosthesis acceptability, a high level of anthropomorphism is also required. It can be addressed by replicating finger number and degrees of freedom, as well as size and weight of the natural hand.
In Figure 5 an attempt of functional scheme of prosthetic system able to satisfy the wishes emerged from the user needs analysis is proposed. It closes the user in the control loop by establishing a bidirectional communication between the user and the prosthetic system and is composed of the following subsystems (1) the interface responsible for the bidirectional communication with the PNS; (2) the control system driving the prosthesis actuators on the basis of proprioceptive and tactile/force sensory information; (3) the sensory system that returns the tactile feedback about the contact with the manipulated object to the prosthesis embedded control as well as to the amputee, through the bidirectional interface.
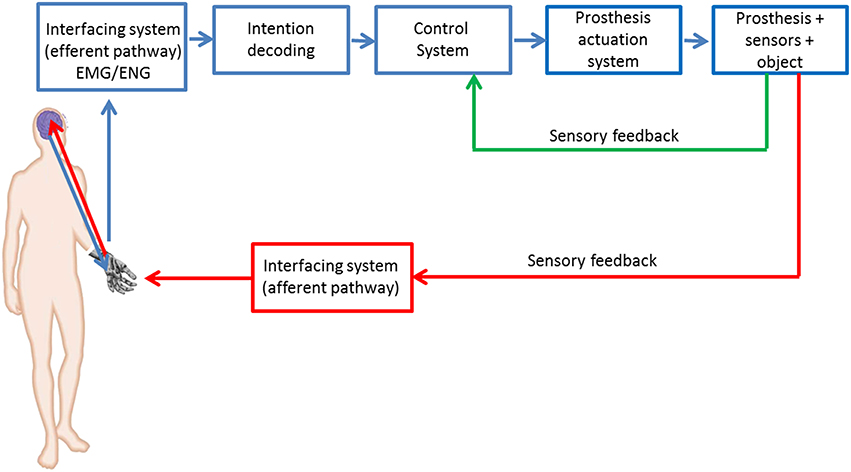
Figure 5. Block scheme of the PNS-based control of a prosthetic system .
The interface with the PNS relies on the acquisition of EMG or ENG signals. In fact, the possibility to perform grasping configurations with low fatigue and more natural and intuitive control makes the EMG-based interfacing system the preferred one as regards the efferent pathway ( Weaver et al., 1988 ). More recently, also ENG signals ( Schultz and Kuiken, 2011 ) have been taken into account for controlling a prosthesis, but this approach is still at research level.
The control system drives the prosthesis actuators starting from the amputee intentions of movement decoded by means of the muscular or neural interfacing system ( Schultz and Kuiken, 2011 ). In order to meet the patient requirements on prosthesis control (in terms of applied force, slippage prevention and movement execution), the control subsystem will receive in input both the patient intention (from EMG/ENG) and the data from a sensory system embedded into the prosthesis.
The needs analysis of upper limb prosthesis also reveals the necessity to provide the prosthetic hand with tactile sensorization for the twofold purpose of performing a force control with the prosthesis and returning force/tactile sensation to the user on the afferent pathway.
Over the years different techniques have been proposed for eliciting tactile sensations ( Antfolk et al., 2013 ; Schofield et al., 2014 ) by means of invasive or non-invasive interfaces.
Skin stimulation through mechanical vibration, i.e., vibrotactile feedback, is the most used non-invasive technique to provide tactile information during grasping tasks with prostheses, because of the small size and weight ( Schofield et al., 2014 ). The electrotactile sensory substitution consists in stimulating the skin by means of local electrical current. Despite the reduced power consumption and the faster response with respect to vibrotactile technique, the electrotactile feedback does not allow perceiving isolated sensations in a specific task. Prosthesis users often manifest adaptation to vibrotactile and electrotactile stimulation. Moreover, both techniques may cause problems in the integration with myoelectric prostheses by contaminating the myoelectric control signals ( Schofield et al., 2014 ). Mechanotactile feedback makes use of tactors to provide a pressure on the skin. Studies in able-bodied subjects demonstrated the possibility to provide various graduated and discriminated levels of force. Although the mechanotactile feedback has higher spatial discrimination than vibrotactile feedback, the systems necessary to furnish pressure on the skin are typically heavier and larger than other feedback methods.
The electrical stimulation of the peripheral nerve, i.e., neural stimulation, offers the advantage of providing a more natural feedback on the afferent pathway, and potentially recovering the bidirectional communication between the prosthesis and the amputee PNS, using neural electrodes (e.g., cuff or intraneural). Despite the invasiveness, this technique allows selectively eliciting various tactile sensations. In recent studies ( Rossini et al., 2010 ; Ortiz-Catalan et al., 2014 ; Raspopovic et al., 2014 ; Tan et al., 2014 ) it has been demonstrated the possibility to neurally elicit tactile sensations, for example of pressure or vibration, on the subjects involved in the studies. In particular, in Rossini et al. (2010) the possibility of controlling a prosthetic hand by means of efferent neural signals acquired with intraneural electrodes has been demonstrated; in Raspopovic et al. (2014) , a first evidence of bidirectional control (intended as efferent and afferent paths closed on the patient) of a prosthetic hand has been developed: the hand control has been performed via EMG sensors and the sensory feedback has been elicited by means of invasive neural interfaces. Electrical stimulation of the nerves for eliciting tactile sensations has also been investigated in Ortiz-Catalan et al. (2014) . Cuff electrodes have been used in Tan et al. (2014) with the aim of demonstrating that perceived sensations and perceived areas can be modulated by changing pulses parameters also in chronic implants (i.e., for long period of time).
This paper has provided a literature review on needs and priorities of prostheses users in order to identify the requirements of a prosthetic system. The performed analysis makes it also possible to identify a list of factors necessary to assess user demands on prostheses:
• demographic/personal factors: gender, education level, marital status, ethnicity, occupational status before and after amputation, hobbies/sports;
• limb loss-related factors: time from limb loss, age at limb loss, limb loss reason, side and level of limb loss, unilateral/bilateral loss;
• prosthesis-related factors: type and number of used prostheses, prosthesis satisfaction related to appearance, comfort, control, reliability, glove durability, dexterity, donning/doffing, noise level, weight, cost of the prosthesis and of its maintenance, speed of opening and closing, grip strength, wrist control, ability to coordinate multiple joints, prosthesis related needs, wearing time, prosthesis usefulness, desired prosthesis improvement;
• prosthesis functionality: since the word “function” can be interpreted in different ways (i.e., to define the user ability to perform ADLs or to characterize the device performance, such as speed or grip strength), the terminology in the questionnaires should be better defined and explained also carrying out examples. In this paper the device performance is included in the prosthesis-related factors while grasping configurations, manipulation tasks and ADLs the user is able to perform, so as the physical effort needed to use the device, should be included in prosthesis functionality factors;
• rehabilitation factors: prosthesis training (if the user ever had prosthesis training, duration of the training, if he/she perceived advantages after training), follow-up. As outlined in Østlie et al. (2012) , individualized prosthesis training should be mandatory in order to improve the prosthesis use.
In order to better compare the results obtained in the studies performed by different research groups, the introduction of a common evaluation scale regarding prosthetic usefulness, such as Likert scale, should be considered.
An in-depth analysis of bilateral prosthesis user needs and priorities should be carried out. Despite the analyzed studies typically involved both unilateral and bilateral users, the presented results are more related to unilateral users because more representative of the statistic sample. The data related to bilateral users require to be analyzed separately from unilateral data in order to correctly assess their feeling with prostheses. The unilateral users typically employ the prosthetic hand as assistive device of the intact limb meanwhile the bilateral users depend significantly more on the device. As pointed out in Østlie et al. (2012) the bilateral users pay more attention, in comparison with unilateral users, to activities related to personal hygiene, such as using a water tap, combing one's hair and blowing one's nose, that typically unilateral users perform with the intact limb.
Conclusions and Future Perspectives
This work contributes to review the literature on the needs of upper limb prosthetic users and extracts the main requirements to be satisfied by the prosthetic systems in order to improve their acceptability and usefulness. The analysis has been focused on seven recent studies, Kyberd and Hill (2007) , Biddiss et al. (2007) , Jang et al. (2011) , Pylatiuk et al. (2007) , Østlie et al. (2012) , Bouffard et al. (2012) , and Lucchetti et al. (2015) which represent the most exhaustive ones in the literature and also include the results of previous studies, about level of satisfaction, needs and preferences of patients wearing upper limb prostheses.
In the proposed literature analysis special attention has been devoted to the comparative analysis of myoelectric, electric, body-powered and passive upper limb prostheses. In particular, for each study the type of prosthesis, level of limb loss, population, answered questions and percentage of response have been reported.
The thorough investigation of the patient needs reveals the requirements the prosthetic system of the future should meet. They can be summarized as follows:
1. To allow the execution of the daily life tasks more important to patients, ensuring the realization of the grasping and manipulation operations identified in Section Needs Analysis of Upper LIMB Prosthesis Users;
2. To integrate into the prosthesis a tactile sensorization system made of contact, sliding, temperature and force sensors;
3. To provide a control system able to command hand actuators independently managing position and force exerted by the fingers on the objects. The role of the visual feedback should be then lightened giving more importance to sensory feedback;
4. To increase the number of grasp types and to move toward manipulation tasks related to everyday life activities (feeding, dressing, cleanliness);
5. To increase the dexterity of the prosthesis enlarging the number of controlled degrees of freedom.
A possible, but not the only one, functional scheme of a prosthetic system (Figure 5 ) able to satisfy the emerged user wishes should be able to communicate in a bidirectional way with the PNS with both aims of controlling in a stable and dexterous way the hand prosthesis and of providing the amputee the tactile sensation related to the task she/he executes.
Furthermore, a list of factors necessary to assess user demands on prostheses has been identified.
In conclusion, despite the great progress made over the years in prosthetic field, this paper reveals the necessity to improve the hand control in order to assure tasks of daily living and to integrate the sensory feedback so as to restore the amputee sensations related to the interaction with the environment.
Author Contributions
FC and AC designed the paper, analyzed the literature and wrote the paper; RS, AD, AGC collaborated during the literature analysis; EG and LZ designed the paper and supervised the writing. All the authors read and approved the manuscript.
This work was supported partly by the Italian Institute for Labour Accidents (INAIL) in the framework of the PPR 2 project (CUP: E58C13000990001), partly by the Italian Ministry of Instruction, University and Research in the framework of the PRIN HANDBOT project (CUP: B81J12002680008) and partly by the Italian Ministry of Health in the framework of the NEMESIS project (CUP: C81J12000380001).
Conflict of Interest Statement
The authors declare that the research was conducted in the absence of any commercial or financial relationships that could be construed as a potential conflict of interest.
1. ^ http://www.salute.gov.it/ricoveriOspedalieri/ric_informazioni/default.jsp
2. ^ http://www.nasdab.co.uk/
3. ^ COAPT Complete control, 2014 Available: http://www.coaptengineering.com/
4. ^ http://www.touchbionics.com/
5. ^ http://www.bebionic.com
6. ^ http://www.living-with-michelangelo.com/home/ <
Antfolk, C., D'Alonzo, M., Rosen, B., Lundborg, G., Sebelius, F., and Cipriani, C. (2013). Sensory feedback in upper limb prosthetics. Expert Rev. Med. Devices. 10, 45–54. doi: 10.1586/erd.12.68
PubMed Abstract | CrossRef Full Text | Google Scholar
Atkins, D. J., Heard, D. C. Y., and Donovan, W. H. (1996). Epidemiologic overview of individuals with upper limb loss and their reported research priorities. J. Prosthet. Orthot. 8, 2–11. doi: 10.1097/00008526-199600810-00003
CrossRef Full Text | Google Scholar
Atzori, M., and Muller, H. (2015). Control capabilities of myoelectric robotic prostheses by hand amputees: a scientific research and market overview. Front. Syst. Neurosci. 9:162. doi: 10.3389/fnsys.2015.00162
Belter, J. T., Segil, J. L., Dollar, A. M., and Weir, R. F. (2013). Mechanical design and performance specifications of anthropomorphic prosthetic hands: a review. J. Rehabil. Res. Dev. 50, 599–618. doi: 10.1682/JRRD.2011.10.0188
Biddiss, E. A., and Chau, T. T. (2007). Upper limb prosthesis use and abandonment: a survey of the last 25 years. Prosthet. Orthot. Int. 31, 236–257. doi: 10.1080/03093640600994581
Biddiss, E., Beaton, D., and Chau, T. (2007). Consumer design priorities for upper limb prosthetics. Disabil. Rehabil. Assist. Technol. 2, 346–357. doi: 10.1080/17483100701714733
Blough, D. K., Hubbard, S., McFarland, L. V., Smith, D. G., Gambel, J. M., and Reiber, G. E. (2010). Prosthetic cost projections for service members with major limb loss from Vietnam and OIF/OEF. J. Rehabil. Res. Dev. 47, 387–402. doi: 10.1682/JRRD.2009.04.0037
Bouffard, J., Vincent, C., Boulianne, E., Lajoie, S., and Mercier, C. (2012). Interactions between the phantom limb sensations, prosthesis use, and rehabilitation as seen by amputees and health professionals. J. Prosthet. Orthot. 24, 25–33. doi: 10.1097/JPO.0b013e318240d171
Castellini, C., Artemiadis, P., Wininger, M., Ajoudani, A., Alimusaj, M., Bicchi, A., et al. (2014). Proceedings of the first workshop on peripheral machine interfaces: going beyond traditional surface electromyography. Front. Neurorobot. 8:22. doi: 10.3389/fnbot.2014.00022
Cho, E., Chen, R., Merhi, L.-K., Xiao, Z., Pousett, B., and Menon, C. (2016). Force myography to control robotic upper extremity prostheses: a feasibility study. Front. Bioeng. Biotechnol. 4:18. doi: 10.3389/fbioe.2016.00018
Clement, R. G. E., Bugler, K. E., and Oliver, C. W. (2011). Bionic prosthetic hands: a review of present technology and future aspirations. Surgeon 9, 336–340. doi: 10.1016/j.surge.2011.06.001
Cloutier, A., and Yang, J. (2013). Design, control, and sensory feedback of externally powered hand prostheses: a literature review. Crit. Rev. Biomed. Eng. 41, 161–181. doi: 10.1615/CritRevBiomedEng.2013007887
Davalli, A., and Sacchetti, R. (2009). “Le protesi di Arto,” in Trattato di Medicina Riabilitativa – cap.25 (Naples: Idelson Gnocchi).
Dhillon, G. S., and Horch, K. W. (2005). Direct neural sensory feedback and control of a prosthetic arm. IEEE Trans. Neural Syst. Rehabil. Eng. 13, 468–472. doi: 10.1109/TNSRE.2005.856072
Dhillon, G. S., Lawrence, S. M., Hutchinson, D. T., and Horch, K. W. (2004). Residual function in peripheral nerve stumps of amputees: implications for neural control of artificial limbs. J. Hand Surg. Am . 29, 605–615. doi: 10.1016/j.jhsa.2004.02.006
Dohnalek, P., Gajdos, P., and Peterek, T. (2013). “Human activity recognition on raw sensors data via sparse approximation,” in Proceedings of the 36th International Conference on Telecommunications and Signal Processing (Rome), 700–703.
Engdahl, S. M., Christie, B. P., Kelly, B., Davis, A., Chestek, C. A., and Gates, D. H. (2015). Surveying the interest of individuals with upper limb loss in novel prosthetic control techniques. J. Neuroeng. Rehabil. 12:53. doi: 10.1186/s12984-015-0044-2
Fougner, A., Stavdahl, O., Kyberd, P. J., Losier, Y. G., and Parker, P. (2012). Control of upper limb prostheses: terminology and proportional myoelectric control: a review. IEEE Trans. Neural Syst. Rehabil. Eng. 20, 663–677. doi: 10.1109/TNSRE.2012.2196711
Fraser, C. (1993). A survey of users of upper limb prostheses. Br. J. occup. Ther. 56, 166–168. doi: 10.1177/030802269305600503
Fraser, C. M. (1998). An evaluation of the use made of cosmetic and functional prostheses by unilateral upper limb amputees. Prosthet. Orthot. Int. 22, 216–223.
PubMed Abstract | Google Scholar
Frontera, W. R., and Silver, J. K. (2014). Fondamenti di Medicina Fisica e Riabilitativa . Rome: Verducci Editore.
Fumero, R., and Costantino, M. L. (2001). “Organi artificiali,” in Storia della Bioingegneria , ed Patron (Bologna), 341–365.
PubMed Abstract
Gonzales, D. S., and Castellini, C. (2013). A realistic implementation of ultrasound imaging as a human-machine interface for upper limb amputees. Front. Neurorobot. 7:17. doi: 10.3389/fnbot.2013.00017
PubMed Abstract | CrossRef Full Text
Heckathorne, C. W. (1994). “Expert analysis of the TIRR National Upper limb Amputee Database,” in Archived at The Institute for Rehabilitation and Research in Houston (Houston).
Google Scholar
Heger, H., Millstein, S., and Hunter, G. A. (1985). Electrically powered prostheses for the adult with an upper limb amputation. J. Bone Joint Surg. 67B, 278–281.
Hijjawi, J. B., Kuiken, T. A., Lipschutz, R. D., Miller, L. A., Stubblefield, K. A., and Dumanian, G. A. (2006). Improved myoelectric prosthesis control accomplished using multiple nerve transfers. Plast. Reconstr. Surg. 118, 1573–1578. doi: 10.1097/01.prs.0000242487.62487.fb
Jang, C. H., Yang, H. S., Yang, H. E., Lee, S. Y., Kwon, J. W., Yun, B. D., et al. (2011). A survey on activities of daily living and occupations of upper extremity amputees. Ann. Rehabil. Med. 35, 907–921. doi: 10.5535/arm.2011.35.6.907
Jiang, N., and Farina, D. (2014). Myoelectric control of upper limb prosthesis: current status, challenges and recent advances. Front. Neuroeng. Conference Abstract: MERIDIAN 30M Workshop . doi: 10.3389/conf.fneng.2014.11.00004. [Epub ahead of print].
Kejlaa, G. H. (1993). Consumer concerns and the functional value of prostheses to upper limb amputees. Prosthet. Orthot. Int. 17, 157–163. doi: 10.3109/03093649309164376
Østlie, K., Lesjø, I. M., Franklin, R. J., Garfelt, B., Skjeldal, O. H., and Magnus, P. (2012). Prosthesis use in adult acquired major upper-limb amputees: patterns of wear, prosthetic skills and the actual use of prostheses in activities of daily life. Disabil. Rehabil. Assist. Technol. 7, 479–493. doi: 10.3109/17483107.2011.653296
Kyberd, P. J., and Hill, W. (2007). Survey of upper limb prosthesis users in sweden and the United Kingdom. J. Prosthet. Orthot. 19, 55–66. doi: 10.1177/0309364611409099
Li, N., Yang, D., Jiang, L., Liu, H., and Cai, H. (2012). Combined use of FSR sensor array and SVM classifier for finger motion recognition based on pressure distribution map. J. Bionic Eng. 9, 39–47. doi: 10.1016/S1672-6529(11)60095-4
Lindner, H., Nätterlund, B. S., and Hermansson, L. M. N. (2010). Upper limb prosthetic outcome measures: review and content comparison based on international classification of functioning, disability and health. Prosthet. Orthot. Int . 34, 109–128. doi: 10.3109/03093641003776976
Lucchetti, M., Cutti, A. G., Verni, G., Sacchetti, R., and Rossi, N. (2015). Impact of michelangelo prosthetic hand: findings from a crossover longitudinal study. J. Rehabil. Res. Dev. 52, 605–618. doi: 10.1682/JRRD.2014.11.0283
Miller, L. A., Stubblefield, K. A., Lipschutz, R. D., Lock, B. A., and Kuiken, T. A. (2008). Improved myoelectric prosthesis control using targeted reinnervation surgery: a case series. IEEE Trans. Neural Syst. Rehabil. Eng. 16, 46–50. doi: 10.1109/TNSRE.2007.911817
Navarro, X., Krueger, T. B., Lago, N., Micera, S., Stieglitz, T., and Dario, P. (2005). A critical review of interfaces with the peripheral nervous system for te control of neuroprostheses and hybrid bionic systems. J. Peripher. Nerv. Syst. 10, 229–258. doi: 10.1111/j.1085-9489.2005.10303.x
Ortiz-Catalan, M., Hakansson, B., and Branemark, R. (2014). An osseointegrated human-machine gateway for long-term sensory feedback and motor control of artificial limbs. Sci. Transl. Med. 6:257re6. doi: 10.1126/scitranslmed.3008933
Pasquina, P. F., Evangelista, M., Carvalho, A. J., Lockhart, J., Griffin, S., Nanos, G., et al. (2015). First-in-man demonstration of a fully implanted myoelectric sensors system to control an advanced electromechanical prosthetic hand. J. Neurosci. Methods 244, 85–93. doi: 10.1016/j.jneumeth.2014.07.016
Peerdeman, B., Boere, D., Witteveen, H. J. B., Hermens, H. J., Stramigioli, S., Rietman, J. S., et al. (2011). Myoelectric forearm prostheses: state of the art from a user-centered perspective. J. Rehabil. Res. Dev. 48, 719–737. doi: 10.1682/JRRD.2010.08.0161
Polasek, K. H., Hoyen, H. A., Keith, M. W., Kirsch, R. F., and Tyler, D. J. (2009). Stimulation stability and selectivity of chronically implanted multicontact nerve cuff electrodes in the human upper extremity. IEEE Trans. Neural Syst. Rehabil. Eng. 17, 428–437. doi: 10.1109/TNSRE.2009.2032603
Pylatiuk, C., Schultz, S., and Doderlein, L. (2007). Results on internet survey of myoelectric prosthetic hand users. Prosthet. Orthot. Int. 31, 362–370. doi: 10.1080/03093640601061265
Raspopovic, S., Capogrosso, M., Petrini, F. M., Bonizzato, M., Rigosa, J., Di Pino, G., et al. (2014). Restoring natural sensory feedback in real-time bidirectional hand prostheses. Sci. Transl. Med. 6:222ra19. doi: 10.1126/scitranslmed.3006820
Rossini, P. M., Micera, S., Benvenuto, A., Carpaneto, J., Cavallo, G., Citi, L., et al. (2010). Double nerve intraneural interface implant on a human amputee for robotic hand control. Clin. Neurophysiol. 121, 777–783. doi: 10.1016/j.clinph.2010.01.001
Schofield, J. S., Evans, K. R., Carey, J. P., and Hebert, J. S. (2014). Applications of sensory feedback in motorized upper extremity prosthesis: a review. Expert Rev. Med. Devices 11, 499–511. doi: 10.1586/17434440.2014.929496
Schultz, A. E., and Kuiken, T. A. (2011). Neural interfaces for control of upper limb prostheses: the state of the art and future possibilities. Phys. Med. Rehabil. 3, 55–67. doi: 10.1016/j.pmrj.2010.06.016
Scott, R. N., and Parker, P. A. (1988). Myoelectric prostheses: state of the art. J. Med. Eng. Technol. 12, 143–151. doi: 10.3109/03091908809030173
Tan, D. W., Schiefer, M. A., Keith, M. W., Anderson, J. R., Tyler, J., and Tyler, D. J. (2014). A neural interface provides long-term stable natural touch perception. Sci. Transl. Med. 6:257ra138. doi: 10.1126/scitranslmed.3008669
Van Lunteren, A., Van Lunteren-Gerritsen, G. N. M., Stassen, N. C., and Zuithoff, M. J. (1983). A field evaluation of arm prostheses for unilateral amputees. Prosthet. Orthot. Int. 7, 141–151. doi: 10.3109/03093648309166586
Weaver, S. A., Lange, L. R., and Vogts, V. M. (1988). Comparison of myoelectric and conventional prostheses for adolescent amputees. Br. J. Occup. Ther. 42, 87–91. doi: 10.5014/ajot.42.2.87
Wininger, M., Kim, N. H., and Craelius, W. (2008). Pressure signature of fore-arm as predictor of grip force. J. Rehabil. Res. Dev. 45, 883–892. doi: 10.1682/JRRD.2007.11.0187
Wright, T. W., Hagen, A. D., and Wood, M. B. (1995). Prosthetic usage in major upper extremity amputations. J. Hand Surg. 20A, 619–622. doi: 10.1016/S0363-5023(05)80278-3
Ziegler-Graham, K., MacKensie, E. J., Ephraim, P. L., Travison, T. G., and Brookmeyer, R. (2008). Estimating the prevalence of limb loss in the United States: 2005 to 2050. Arch. Phys. Med. Rehabil. 89, 422–429. doi: 10.1016/j.apmr.2007.11.005
Keywords: upper limb prosthesis, patient needs, grasping, prosthesis requirements, PNS-based prosthesis
Citation: Cordella F, Ciancio AL, Sacchetti R, Davalli A, Cutti AG, Guglielmelli E and Zollo L (2016) Literature Review on Needs of Upper Limb Prosthesis Users. Front. Neurosci . 10:209. doi: 10.3389/fnins.2016.00209
Received: 03 November 2015; Accepted: 25 April 2016; Published: 12 May 2016.
Reviewed by:
Copyright © 2016 Cordella, Ciancio, Sacchetti, Davalli, Cutti, Guglielmelli and Zollo. This is an open-access article distributed under the terms of the Creative Commons Attribution License (CC BY) . The use, distribution or reproduction in other forums is permitted, provided the original author(s) or licensor are credited and that the original publication in this journal is cited, in accordance with accepted academic practice. No use, distribution or reproduction is permitted which does not comply with these terms.
*Correspondence: Francesca Cordella, [email protected]
† These authors have contributed equally to this work.
Disclaimer: All claims expressed in this article are solely those of the authors and do not necessarily represent those of their affiliated organizations, or those of the publisher, the editors and the reviewers. Any product that may be evaluated in this article or claim that may be made by its manufacturer is not guaranteed or endorsed by the publisher.
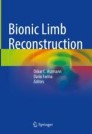
Bionic Limb Reconstruction pp 197–214 Cite as
Principles of Occupational and Physical Therapy in Upper Limb Amputations
- Diane J. Atkins 3 &
- Agnes Sturma 4 , 5
- First Online: 05 January 2021
875 Accesses
1 Citations
The unique background, education, and training of occupational and physical therapists enable them to have a profound influence on an individual with upper limb loss. Understanding the many phases of amputee prosthetic rehabilitation is required in order to meet the functional needs of individuals fit with body-powered and myoelectric prostheses. Thoughtfully designed protocols include interventions before surgical treatment, after surgery, pre-prosthetic interventions, and prosthetic training with the aim to enable the amputee to achieve an optimal functional outcome. These protocols are designed to support the individual with upper limb loss in regaining their independence and are modified dependent on the individual life situations and the prosthetic device used. In view of the emerging advances in upper limb surgery and technology, a collaborative relationship with the entire team of surgeons, prosthetists, and rehabilitation professionals is more important than ever as we prepare each patient to reach their maximum potential.
- Amputee rehabilitation
- Prosthetic training
- Controls training
- Targeted muscle reinnervation
This is a preview of subscription content, log in via an institution .
Buying options
- Available as PDF
- Read on any device
- Instant download
- Own it forever
- Available as EPUB and PDF
- Compact, lightweight edition
- Dispatched in 3 to 5 business days
- Free shipping worldwide - see info
- Durable hardcover edition
Tax calculation will be finalised at checkout
Purchases are for personal use only
Aszmann OC, et al. Bionic reconstruction to restore hand function after brachial plexus injury: a case series of three patients. Lancet. 2015;385(9983):2183–9.
Article PubMed Google Scholar
Aszmann OC, et al. Elective amputation and bionic substitution restore functional hand use after critical soft tissue injuries. Sci Rep. 2016;6:34960.
Article CAS PubMed PubMed Central Google Scholar
Atkins D. The upper extremity prosthetic prescription: conventional or electric components . Phys Disabil Spec Int OT Newslett. 1987;10(2).
Google Scholar
Atkins DJ. Adult upper-limb prosthetic training. In: Atkins DJ, Meier RH III, editors. Comprehensive management of the upper-limb amputee. New York: Springer; 1989.
Batsford S, Ryan CG, Martin DJ. Non-pharmacological conservative therapy for phantom limb pain: a systematic review of randomized controlled trials. Physiother Theory Pract. 2017;33(3):173–83.
Bergmeister KD, et al. Broadband prosthetic interfaces: combining nerve transfers and implantable multichannel EMG technology to decode spinal motor neuron activity . Front Neurosci. 2017;11(421).
Biddiss EA, Chau TT. Multivariate prediction of upper limb prosthesis acceptance or rejection. Disabil Rehabil Assist Technol. 2008;3(4):181–92.
Bouwsema H, van der Sluis CK, Bongers RM. Changes in performance over time while learning to use a myoelectric prosthesis. J Neuroeng Rehabil. 2014;11(1):16.
Article PubMed PubMed Central Google Scholar
Carey SL, et al. Compensatory movements of transradial prosthesis users during common tasks. Clin Biomech (Bristol, Avon). 2008;23(9):1128–35.
Article Google Scholar
Carey SL, Lura DJ, Highsmith MJ. Differences in myoelectric and body-powered upper-limb prostheses: systematic literature review. J Rehabil Res Dev. 2015;52(3):247–62.
Carlsen BT, Prigge P, Peterson J. Upper extremity limb loss: functional restoration from prosthesis and targeted reinnervation to transplantation. J Hand Ther. 2014;27(2):106–13; quiz 114.
Cordella F, et al. Literature review on needs of upper limb prosthesis users. Front Neurosci. 2016;10:209.
Dickstein R, Deutsch JE. Motor imagery in physical therapist practice. Phys Ther. 2007;87(7):942–53.
Durance JP, O’Shea BJ. Upper limb amputees: a clinic profile. Int Disabil Stud. 1988;10(2):68–72.
Article CAS PubMed Google Scholar
Ehrsson H, Geyer S, Naito E. Imagery of voluntary movement of fingers, toes, and tongue activates corresponding body-part-specific motor representations. J Neurophysiol. 2003;90(5):3304–16.
Ezendam D, Bongers RM, Jannink MJA. Systematic review of the effectiveness of mirror therapy in upper extremity function. Disabil Rehabil. 2009;31(26):2135–49.
Ferraro F, et al. Diagnosis and treatment of pain in plexopathy, radiculopathy, peripheral neuropathy and phantom limb pain. Evidence and recommendations from the Italian Consensus Conference on Pain on Neurorehabilitation. Eur J Phys Rehabil Med. 2016;52:855–66.
PubMed Google Scholar
Fu Q, Santello M. Improving fine control of grasping force during hand-object interactions for a soft synergy-inspired myoelectric prosthetic hand. Front Neurorobot. 2017;11:71.
Hruby LA, et al. Algorithm for bionic hand reconstruction in patients with global brachial plexopathies. J Neurosurg. 2017;127(5):1163–71.
Jackson PL, Lafleur MF, Malouin F, Richards C, Doyon J. Potential role of mental practice using motor imagery in neurologic rehabilitation. Arch Phys Med Rehabil. 2001;82(8):1133–41.
Jones LE, Davidson JH. Save that arm: a study of problems in the remaining arm of unilateral upper limb amputees. Prosthetics Orthot Int. 1999;23(1):55–8.
Article CAS Google Scholar
Jonsson S, Caine-Winterberger K, Branemark R. Osseointegration amputation prostheses on the upper limbs: methods, prosthetics and rehabilitation. Prosthetics Orthot Int. 2011;35(2):190–200.
Kuiken TA, et al. The use of targeted muscle reinnervation for improved myoelectric prosthesis control in a bilateral shoulder disarticulation amputee. Prosthetics Orthot Int. 2004;28(3):245–53.
Kuiken TA, et al. Targeted muscle reinnervation for the upper and lower extremity. Tech Orthop. 2017;32(2):109–16.
Malone JM, et al. Immediate, early, and late postsurgical management of upper-limb amputation. J Rehabil Res Dev. 1984;21(1):33–41.
CAS PubMed Google Scholar
Miguelez J, Conyers D, Keams N. Using meaningful data to optimize the quality of life for persons with upper limb amputation. In: American Academy of Orthotists & Prosthetists annual meeting; 2017.
Moseley GL. Graded motor imagery for pathologic pain: a randomized controlled trial. Neurology. 2006;67(12):2129–34.
Ortiz-Catalan M, et al. Phantom motor execution facilitated by machine learning and augmented reality as treatment for phantom limb pain: a single group, clinical trial in patients with chronic intractable phantom limb pain. Lancet. 2016;388(10062):2885–94.
Østlie K. Evidence-based guideline for rehabilitation after acquired upper-limb loss in Norway [Norwegian]. 2016. www.magicapp.org/public/guideline/Jn3zaL . Accessed 30 Mar 2016.
Ostlie K, et al. Assessing physical function in adult acquired major upper-limb amputees by combining the Disabilities of the Arm, Shoulder and Hand (DASH) Outcome Questionnaire and clinical examination. Arch Phys Med Rehabil. 2011;92(10):1636–45.
Prahm C, et al. PlayBionic: game-based interventions to encourage patient engagement and performance in prosthetic motor rehabilitation. PM&R. 2018;10(11):1252–60.
Ramachandran VS, Rogers-Ramachandran D. Synaesthesia in phantom limbs induced with mirrors. Proc Biol Sci. 1996;263(1369):377–86.
Rossi M, et al. HapPro: a wearable haptic device for proprioceptive feedback. IEEE Trans Biomed Eng. 2019;66(1):138–49.
Saikia A, et al. Recent advancements in prosthetic hand technology. J Med Eng Technol. 2016;40(5):255–64.
Salminger S, et al. [Prosthetic reconstruction of the upper extremity] . Unfallchirurg. 2016;119(5):408–13.
Salminger S, et al. [Prosthetic reconstruction in high amputations of the upper extremity] . Orthopade. 2015;44(6):413–8.
Schofield JS, et al. Applications of sensory feedback in motorized upper extremity prosthesis: a review. Expert Rev Med Devices. 2014;11(5):499–511.
Schweitzer W, Thali MJ, Egger D. Case-study of a user-driven prosthetic arm design: bionic hand versus customized body-powered technology in a highly demanding work environment. J Neuroeng Rehabil. 2018;15(1):1.
Simon AM, Lock BA, Stubblefield KA. Patient training for functional use of pattern recognition-controlled prostheses. J Prosthet Orthot. 2012;24(2):56–64.
Smith D, Michael J, Bowker J. Atlas of amputations and limb deficiencies. Rosemont, IL: American Academy of Orthopaedic Surgeons; 2004.
Smurr LM, et al. Managing the upper extremity amputee: a protocol for success. J Hand Ther. 2008;21(2):160–76.
Stubblefield KA, et al. Occupational therapy protocol for amputees with targeted muscle reinnervation. J Rehabil Res Dev. 2009;46(4):481–8.
Sturma A, et al. Recommendation for therapy after targeted muscle reinnervation. In: University of New Brunswick’s myoelectric controls/powered prosthetics symposium. New Brunswick: Institute of Biomedical Engineering, University of New Brunswick; 2014.
Sturma A, et al. Rehabilitation of upper extremity nerve injuries using surface EMG biofeedback: protocols for clinical application. Front Neurosci. 2018;12:906.
Sturma A, Salminger S, Aszmann O. Proximale Amputationen des Armes: Technische, chirurgische und handtherapeutische Möglichkeiten. Zeitschrift für Handtherapie. 2018;21(1):18–25.
Vujaklija I, Farina D. 3D printed upper limb prosthetics. Expert Rev Med Devices. 2018;15(7):505–12.
Wakolbinger R, et al. Home based tactile discrimination training (TDT) reduces phantom limb pain. Pain Pract. 2017;18(6):709–15.
Weeks DLP, Anderson DIP, Wallace SAP. The role of variability in practice structure when learning to use an upper-extremity prosthesis. JPO J Prosthet Orthot. 2003;15(3):84–92.
Download references
Author information
Authors and affiliations.
Department of Physical Medicine and Rehabilitation, Baylor College of Medicine, Houston, TX, USA
Diane J. Atkins
Clinical Laboratory for Bionic Extremity Reconstruction, Medical University of Vienna, Vienna, Austria
Agnes Sturma
Department of Bioengineering, Imperial College London, London, UK
You can also search for this author in PubMed Google Scholar
Editor information
Editors and affiliations.
Division of Plastic and Reconstructive Surgery, Medical University of Vienna, Vienna, Austria
Oskar C. Aszmann
Dario Farina
Rights and permissions
Reprints and permissions
Copyright information
© 2021 Springer Nature Switzerland AG
About this chapter
Cite this chapter.
Atkins, D.J., Sturma, A. (2021). Principles of Occupational and Physical Therapy in Upper Limb Amputations. In: Aszmann, O.C., Farina, D. (eds) Bionic Limb Reconstruction. Springer, Cham. https://doi.org/10.1007/978-3-030-60746-3_20
Download citation
DOI : https://doi.org/10.1007/978-3-030-60746-3_20
Published : 05 January 2021
Publisher Name : Springer, Cham
Print ISBN : 978-3-030-60745-6
Online ISBN : 978-3-030-60746-3
eBook Packages : Medicine Medicine (R0)
Share this chapter
Anyone you share the following link with will be able to read this content:
Sorry, a shareable link is not currently available for this article.
Provided by the Springer Nature SharedIt content-sharing initiative
- Publish with us
Policies and ethics
- Find a journal
- Track your research
Thank you for visiting nature.com. You are using a browser version with limited support for CSS. To obtain the best experience, we recommend you use a more up to date browser (or turn off compatibility mode in Internet Explorer). In the meantime, to ensure continued support, we are displaying the site without styles and JavaScript.
- View all journals
- Explore content
- About the journal
- Publish with us
- Sign up for alerts
- Perspective
- Published: 31 May 2021
Toward higher-performance bionic limbs for wider clinical use
- Dario Farina ORCID: orcid.org/0000-0002-7883-2697 1 ,
- Ivan Vujaklija ORCID: orcid.org/0000-0002-7394-9474 2 ,
- Rickard Brånemark ORCID: orcid.org/0000-0002-1695-3514 3 , 4 ,
- Anthony M. J. Bull ORCID: orcid.org/0000-0002-4473-8264 1 ,
- Hans Dietl 5 ,
- Bernhard Graimann 6 ,
- Levi J. Hargrove 7 , 8 , 9 ,
- Klaus-Peter Hoffmann 10 ,
- He (Helen) Huang 11 , 12 ,
- Thorvaldur Ingvarsson 13 , 14 ,
- Hilmar Bragi Janusson 15 ,
- Kristleifur Kristjánsson 13 ,
- Todd Kuiken 7 , 8 , 9 ,
- Silvestro Micera ORCID: orcid.org/0000-0003-4396-8217 16 , 17 , 18 ,
- Thomas Stieglitz 19 ,
- Agnes Sturma ORCID: orcid.org/0000-0003-2066-1394 1 , 20 ,
- Dustin Tyler ORCID: orcid.org/0000-0002-2298-8510 21 , 22 ,
- Richard F. ff. Weir 23 &
- Oskar C. Aszmann 20
Nature Biomedical Engineering volume 7 , pages 473–485 ( 2023 ) Cite this article
7521 Accesses
91 Citations
170 Altmetric
Metrics details
- Biomedical engineering
- Electromyography – EMG
- Motor control
- Sensorimotor processing
- Translational research
Most prosthetic limbs can autonomously move with dexterity, yet they are not perceived by the user as belonging to their own body. Robotic limbs can convey information about the environment with higher precision than biological limbs, but their actual performance is substantially limited by current technologies for the interfacing of the robotic devices with the body and for transferring motor and sensory information bidirectionally between the prosthesis and the user. In this Perspective, we argue that direct skeletal attachment of bionic devices via osseointegration, the amplification of neural signals by targeted muscle innervation, improved prosthesis control via implanted muscle sensors and advanced algorithms, and the provision of sensory feedback by means of electrodes implanted in peripheral nerves, should all be leveraged towards the creation of a new generation of high-performance bionic limbs. These technologies have been clinically tested in humans, and alongside mechanical redesigns and adequate rehabilitation training should facilitate the wider clinical use of bionic limbs.
This is a preview of subscription content, access via your institution
Access options
Access Nature and 54 other Nature Portfolio journals
Get Nature+, our best-value online-access subscription
24,99 € / 30 days
cancel any time
Subscribe to this journal
Receive 12 digital issues and online access to articles
92,52 € per year
only 7,71 € per issue
Rent or buy this article
Prices vary by article type
Prices may be subject to local taxes which are calculated during checkout
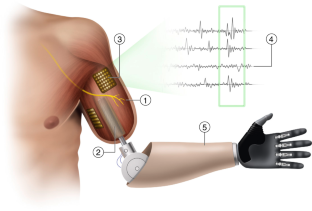
Cordella, F. et al. Literature review on needs of upper limb prosthesis users. Front. Neurosci. 10 , 209 (2016).
Article PubMed Central PubMed Google Scholar
Webster, J. B. et al. Prosthetic fitting, use, and satisfaction following lower-limb amputation: a prospective study. J. Rehabil. Res. Dev. 49 , 1493–1504 (2012).
Article PubMed Google Scholar
Biddiss, E., Beaton, D. & Chau, T. Consumer design priorities for upper limb prosthetics. Disabil. Rehabil. Assist. Technol. 2 , 346–357 (2007).
Kyberd, P. J. & Hill, W. Survey of upper limb prosthesis users in Sweden, the United Kingdom and Canada. Prosthet. Orthot. Int. 35 , 234–241 (2011).
Pylatiuk, C., Schulz, S. & Döderlein, L. Results of an internet survey of myoelectric prosthetic hand users. Prosthet. Orthot. Int. 31 , 362–370 (2007).
Jang, C. H. et al. A survey on activities of daily living and occupations of upper extremity amputees. Ann. Rehabil. Med. 35 , 907–921 (2011).
Fogelberg, D. J., Allyn, K. J., Smersh, M. & Maitland, M. E. What people want in a prosthetic foot. J. Prosthet. Orthot. 28 , 145–151 (2016).
Villa, C. et al. Cross-slope and level walking strategies during swing in individuals with lower limb amputation. Arch. Phys. Med. Rehabil. 98 , 1149–1157 (2017).
Meulenbelt, H., Geertzen, J., Jonkman, M. & Dijkstra, P. Skin problems of the stump in lower limb amputees: 1. A clinical study. Acta Derm. Venereol. 91 , 173–177 (2011).
The Amputee Statistical Database for the United Kingdom 2004/05 (NHS Scotland Information Services Division, 2005); http://www.limbless-statistics.org
Dillingham, T. R., Pezzin, L. E., MacKenzie, E. J. & Burgess, A. R. Use and satisfaction with prosthetic devices among persons with trauma-related amputations: a long-term outcome study. Am. J. Phys. Med. Rehabil. 80 , 563–571 (2001).
Article CAS PubMed Google Scholar
Koc, E. et al. Skin problems in amputees: a descriptive study. Int. J. Dermatol. 47 , 463–466 (2008).
Ding, Z., Jarvis, H. L., Bennett, A. N., Baker, R. & Bull, A. M. Higher knee contact forces might underlie increased osteoarthritis rates in high functioning amputees: a pilot study. J. Orthop. Res. 39 , 850–860 (2021).
Daly, W., Voo, L., Rosenbaum-Chou, T., Arabian, A. & Boone, D. Socket pressure and discomfort in upper-limb prostheses: a preliminary study. JPO J. Prosthet. Orthot. 26 , 99–106 (2014).
Article Google Scholar
Biddiss, E. A. & Chau, T. T. Upper limb prosthesis use and abandonment: a survey of the last 25 years. Prosthet. Orthot. Int. 31 , 236–257 (2007).
Vujaklija, I., Farina, D. & Aszmann, O. New developments in prosthetic arm systems. Orthop. Res. Rev. 8 , 31–39 (2016).
PubMed Central PubMed Google Scholar
Peerdeman, B. et al. Myoelectric forearm prostheses: state of the art from a user-centered perspective. J. Rehabil. Res. Dev. 48 , 719–737 (2011).
Belter, J. T., Segil, J. L., Dollar, A. M. & Weir, R. F. Mechanical design and performance specifications of anthropomorphic prosthetic hands: a review. J. Rehabil. Res. Dev. 50 , 599–618 (2013).
Farina, D. & Aszmann, O. Bionic limbs: clinical reality and academic promises. Sci. Transl. Med. 6 , 257ps12 (2014).
Ning, J., Dosen, S., Muller, K.-R. & Farina, D. Myoelectric control of artificial limbs—is there a need to change focus? IEEE Signal Process. Mag. 29 , 152–150 (2012).
Castellini, C., Bongers, R. M., Nowak, M. & van der Sluis, C. K. Upper-limb prosthetic myocontrol: two recommendations. Front. Neurosci. 9 , 496 (2016).
Bicchi, A. & Sorrentino, R. Dexterous manipulation through rolling. In Proc. 1995 IEEE International Conference on Robotics and Automation 452–457 (IEEE, 1995).
Okamura, A. M., Smaby, N. & Cutkosky, M. R. An overview of dexterous manipulation. In Proc. 2000 IEEE International Conference on Robotics and Automation 255–262 (IEEE, 2000).
Shimoga, K. B. Robot grasp synthesis algorithms: a survey. Int. J. Rob. Res. 15 , 230–266 (1996).
Bicchi, A. Hands for dexterous manipulation and robust grasping: a difficult road toward simplicity. IEEE Trans. Robot. Autom. 16 , 652–662 (2000).
Fishel, J. A. & Loeb, G. E. Sensing tactile microvibrations with the BioTac—comparison with human sensitivity. In 2012 4th IEEE RAS & EMBS International Conference on Biomedical Robotics and Biomechatronics (BioRob) 1122–1127 (IEEE, 2012).
Sewell, P., Noroozi, S., Vinney, J. & Andrews, S. Developments in the trans-tibial prosthetic socket fitting process: a review of past and present research. Prosthet. Orthot. Int. 24 , 97–107 (2000).
Astrom, I. & Stenstrom, A. Effect on gait and socket comfort in unilateral trans-tibial amputees after exchange to a polyurethane concept. Prosthet. Orthot. Int. 28 , 28–36 (2004).
Ortiz-Catalan, M., Brånemark, R., Håkansson, B. & Delbeke, J. On the viability of implantable electrodes for the natural control of artificial limbs: review and discussion. Biomed. Eng. Online 11 , 33 (2012).
Zhou, P. et al. Decoding a new neural–machine interface for control of artificial limbs. J. Neurophysiol. 98 , 2974–2982 (2007).
Scott, R. N. & Parker, P. A. Myoelectric prostheses: state of the art. J. Med. Eng. Technol. 12 , 143–151 (1988).
Lake, C. The evolution of upper limb prosthetic socket design. JPO J. Prosthet. Orthot. 20 , 85–92 (2008).
Potter, M. B. K. et al. Heterotopic ossification following combat-related trauma. J. Bone Joint Surg. Am. 92 , 74–89 (2010).
Brånemark, R., Brånemark, P.-I., Rydevik, B. & Myers, R. R. Osseointegration in skeletal reconstruction and rehabilitation: a review. J. Rehabil. Res. Dev. 38 , 175–181 (2001).
PubMed Google Scholar
Shelton, T. J., Beck, P. J., Bloebaum, R. D. & Bachus, K. N. Percutaneous osseointegrated prostheses for amputees: limb compensation in a 12-month ovine model. J. Biomech. 44 , 2601–2606 (2011).
Jönsson, S., Caine-Winterberger, K. & Brånemark, R. Osseointegration amputation prostheses on the upper limbs: methods, prosthetics and rehabilitation. Prosthet. Orthot. Int. 35 , 190–200 (2011).
Hagberg, K., Branemark, R., Gunterberg, B. & Rydevik, B. Osseointegrated trans-femoral amputation prostheses: prospective results of general and condition-specific quality of life in 18 patients at 2-year follow-up. Prosthet. Orthot. Int. 32 , 29–41 (2008).
Hagberg, K., Häggström, E., Uden, M. & Brånemark, R. Socket versus bone-anchored trans-femoral prostheses: hip range of motion and sitting comfort. Prosthet. Orthot. Int. 29 , 153–163 (2005).
Pitkin, M. Design features of implants for direct skeletal attachment of limb prostheses. J. Biomed. Mater. Res. A 101 , 3339–3348 (2013).
CAS PubMed Central PubMed Google Scholar
Brånemark, R. P., Hagberg, K., Kulbacka-Ortiz, K., Berlin, Ö. & Rydevik, B. Osseointegrated percutaneous prosthetic system for the treatment of patients with transfemoral amputation: a prospective five-year follow-up of patient-reported outcomes and complications. J. Am. Acad. Orthop. Surg. 27 , E743–E751 (2019).
Al Muderis, M., Khemka, A., Lord, S. J., Van de Meent, H. & Frölke, J. P. M. Safety of osseointegrated implants for transfemoral amputees. J. Bone Joint. Surg. 98 , 900–909 (2016).
Ortiz-Catalan, M., Hakansson, B. & Branemark, R. An osseointegrated human-machine gateway for long-term sensory feedback and motor control of artificial limbs. Sci. Transl. Med. 6 , 257re6 (2014).
Mastinu, E., Doguet, P., Botquin, Y., Hakansson, B. & Ortiz-Catalan, M. Embedded system for prosthetic control using implanted neuromuscular interfaces accessed via an osseointegrated implant. IEEE Trans. Biomed. Circuits Syst. 11 , 867–877 (2017).
Ortiz-Catalan, M., Mastinu, E., Sassu, P., Aszmann, O. & Brånemark, R. Self-contained neuromusculoskeletal arm prostheses. New Engl. J. Med. 382 , 1732–1738 (2020).
Matthews, D. J. et al. UK trial of the osseointegrated prosthesis for the rehabilitation for amputees: 1995–2018. Prosthet. Orthot. Int. 43 , 112–122 (2019).
Resnik, L., Benz, H., Borgia, M. & Clark, M. A. Patient perspectives on osseointegration: a national survey of veterans with upper limb amputation. PM&R 11 , 1261–1271 (2019).
Van Nes, C. P. Rotation-plasty for congenital defects of the femur. J. Bone Joint. Surg. Br. 32-B , 12–16 (1950).
Azocar, A. F. et al. Design and clinical implementation of an open-source bionic leg. Nat. Biomed. Eng. 4 , 941–953 (2020).
Goldfarb, M., Lawson, B. E. & Shultz, A. H. Realizing the promise of robotic leg prostheses. Sci. Transl. Med. 5 , 225 (2013).
Grimes, D. L., Flowers, W. C. & Donath, M. Feasibility of an active control scheme for above knee prostheses. J. Biomech. Eng. 99 , 215–221 (1977).
Sup, F., Bohara, A. & Goldfarb, M. Design and control of a powered transfemoral prosthesis. Int. J. Rob. Res. 27 , 263–273 (2008).
Martinez-Villalpando, E. C. & Herr, H. Agonist–antagonist active knee prosthesis: a preliminary study in level-ground walking. J. Rehabil. Res. Dev. 46 , 361 (2009).
Simon, A. M., Hargrove, L. J., Lock, B. A. & Kuiken, T. A. Target achievement control test: evaluating real-time myoelectric pattern-recognition control of multifunctional upper-limb prostheses. J. Rehabil. Res. Dev. 48 , 619–627 (2011).
Young, A. J., Simon, A. M., Fey, N. P. & Hargrove, L. J. Intent recognition in a powered lower limb prosthesis using time history information. Ann. Biomed. Eng. 42 , 631–641 (2014).
Lenzi, T., Sensinger, J., Lipsey, J., Hargrove, L. & Kuiken, T. Design and preliminary testing of the RIC hybrid knee prosthesis. In 37th Annual International Conference of the IEEE Engineering in Medicine and Biology Society (EMBC) 1683–1686 (IEEE, 2015).
Lawson, B. E., Varol, H. A., Huff, A., Erdemir, E. & Goldfarb, M. Control of stair ascent and descent with a powered transfemoral prosthesis. IEEE Trans. Neural Syst. Rehabil. Eng. 21 , 466–473 (2013).
Au, S., Berniker, M. & Herr, H. Powered ankle-foot prosthesis to assist level-ground and stair-descent gaits. Neural Netw. 21 , 654–666 (2008).
Varol, H. A., Sup, F. & Goldfarb, M. Multiclass real-time intent recognition of a powered lower limb prosthesis. IEEE Trans. Biomed. Eng. 57 , 542–551 (2010).
Huang, H. et al. Continuous locomotion-mode identification for prosthetic legs based on neuromuscular–mechanical fusion. IEEE Trans. Biomed. Eng. 58 , 2867–2875 (2011).
Weir, R. F., Heckathorne, C. W. & Childress, D. S. Cineplasty as a control input for externally powered prosthetic components. J. Rehabil. Res. Dev. 38 , 357–363 (2001).
CAS PubMed Google Scholar
Brückner, L. Sauerbruch-Lebsche-Vanghetti cineplasty: the surgical procedure. Orthop. Traumatol. 1 , 90–99 (1992).
Kruit, J. & Cool, J. C. Body-powered hand prosthesis with low operating power for children. J. Med. Eng. Technol. 13 , 129–133 (1989).
Doeringer, J. A. & Hogan, N. Performance of above elbow body-powered prostheses in visually guided unconstrained motion tasks. IEEE Trans. Biomed. Eng. 42 , 621–631 (1995).
Carey, S. L., Lura, D. J. & Highsmith, M. J. Differences in myoelectric and body-powered upper-limb prostheses: systematic literature review. J. Rehabil. Res. Dev. 52 , 247–262 (2015).
Schweitzer, W., Thali, M. J. & Egger, D. Case-study of a user-driven prosthetic arm design: bionic hand versus customized body-powered technology in a highly demanding work environment. J. Neuroeng. Rehabil. 15 , 1 (2018).
Riener, R. The Cybathlon promotes the development of assistive technology for people with physical disabilities. J. Neuroeng. Rehabil. 13 , 49 (2016).
Hargrove, L. J., Simon, A. M., Lipschutz, R., Finucane, S. B. & Kuiken, T. A. Non-weight-bearing neural control of a powered transfemoral prosthesis. J. Neuroeng. Rehabil. 10 , 62 (2013).
Ha, K. H., Varol, H. A. & Goldfarb, M. Volitional control of a prosthetic knee using surface electromyography. IEEE Trans. Biomed. Eng. 58 , 144–151 (2011).
Hargrove, L. J. et al. Intuitive control of a powered prosthetic leg during ambulation. JAMA 313 , 2244–2252 (2015).
Peng, J., Fey, N. P., Kuiken, T. A. & Hargrove, L. J. Anticipatory kinematics and muscle activity preceding transitions from level-ground walking to stair ascent and descent. J. Biomech. 49 , 528–536 (2016).
Zhang, F., Liu, M. & Huang, H. Effects of locomotion mode recognition errors on volitional control of powered above-knee prostheses. IEEE Trans. Neural Syst. Rehabil. Eng. 23 , 64–72 (2015).
Khademi, G., Mohammadi, H. & Simon, D. Gradient-based multi-objective feature selection for gait mode recognition of transfemoral amputees. Sensors 19 , 253 (2019).
Spanias, J. A., Simon, A. M., Finucane, S. B., Perreault, E. J. & Hargrove, L. J. Online adaptive neural control of a robotic lower limb prosthesis. J. Neural Eng. 15 , 016015 (2018).
Article CAS PubMed Central PubMed Google Scholar
Au, S. K., Bonato, P. & Herr, H. An EMG-position controlled system for an active ankle-foot prosthesis: an initial experimental study. In 9th International Conference on Rehabilitation Robotics, ICORR 2005 375–379 (IEEE, 2005).
Zhang, F., Liu, M. & Huang, H. Investigation of timing to switch control mode in powered knee prostheses during task transitions. PLoS ONE 10 , e0133965 (2015).
Stevens, P. M. & Highsmith, M. J. Myoelectric and body power, design options for upper-limb prostheses. J. Prosthet. Orthot. 29 , P1–P3 (2017).
Parker, P., Englehart, K. & Hudgins, B. Myoelectric signal processing for control of powered limb prostheses. J. Electromyogr. Kinesiol. 16 , 541–548 (2006).
Hudgins, B., Parker, P. & Scott, R. N. A new strategy for multifunction myoelectric control. IEEE Trans. Biomed. Eng. 40 , 82–94 (1993).
Graupe, D. & Cline, W. K. Functional separation of EMG signals via ARMA identification methods for prosthesis control purposes. IEEE Trans. Syst. Man Cybern. 5 , 252–259 (1975).
Englehart, K., Hudgin, B. & Parker, P. A. A wavelet-based continuous classification scheme for multifunction myoelectric control. IEEE Trans. Biomed. Eng. 48 , 302–311 (2001).
Hargrove, L. J., Guanglin, L., Englehart, K. B. & Hudgins, B. S. Principal components analysis preprocessing for improved classification accuracies in pattern-recognition-based myoelectric control. IEEE Trans. Biomed. Eng. 56 , 1407–1414 (2009).
Englehart, K. & Hudgins, B. A robust, real-time control scheme for multifunction myoelectric control. IEEE Trans. Biomed. Eng. 50 , 848–854 (2003).
Scheme, E. & Englehart, K. Electromyogram pattern recognition for control of powered upper-limb prostheses: state of the art and challenges for clinical use. J. Rehabil. Res. Dev. 48 , 643–660 (2011).
Ohnishi, K., Weir, R. F. & Kuiken, T. A. Neural machine interfaces for controlling multifunctional powered upper-limb prostheses. Expert Rev. Med. Dev. 4 , 43–53 (2007).
Light, C. M. & Chappell, P. H. Development of a lightweight and adaptable multiple-axis hand prosthesis. Med. Eng. Phys. 22 , 679–684 (2000).
Simon, A. M., Lock, B. A. & Stubblefield, K. A. Patient training for functional use of pattern recognition-controlled prostheses. J. Prosthet. Orthot. 24 , 56–64 (2012).
Hargrove, L., Englehart, K. & Hudgins, B. The effect of electrode displacements on pattern recognition based myoelectric control. In 2006 International Conference of the IEEE Engineering in Medicine and Biology Society 2203–2206 (IEEE, 2006).
Kuiken, T., Miller, L., Turner, K. & Hargrove, L. A comparison of pattern recognition control and direct control of a multiple degree-of-freedom transradial prosthesis. IEEE J. Transl. Eng. Heal. Med. 4 , 2100508 (2016).
Google Scholar
Cipriani, C., Segil, J. L., Birdwell, J. A. & Weir, R. F. Dexterous control of a prosthetic hand using fine-wire intramuscular electrodes in targeted extrinsic muscles. IEEE Trans. Neural Syst. Rehabil. Eng. 22 , 828–836 (2014).
Hahne, J. M., Farina, D., Jiang, N. & Liebetanz, D. A novel percutaneous electrode implant for improving robustness in advanced myoelectric control. Front. Neurosci. 10 , 114 (2016).
Pasquina, P. F. et al. First-in-man demonstration of a fully implanted myoelectric sensors system to control an advanced electromechanical prosthetic hand. J. Neurosci. Methods 244 , 85–93 (2015).
Weir, R. F. et al. Implantable myoelectric sensors (IMESs) for intramuscular electromyogram recording. IEEE Trans. Biomed. Eng. 56 , 159–171 (2009).
Lewis, S. et al. Fully implantable multi-channel measurement system for acquisition of muscle activity. IEEE Trans. Instrum. Meas. 62 , 1972–1981 (2013).
McDonnall, S., Hiatt, S., Crofts, B., Smith, C. & Merrill, D. Development of a wireless multichannel myoelectric implant for prosthesis control. In Proc. Myoelectric Control and Upper Limb Prosthesis Symposium (MEC 2017) 21 (2017).
Graczyk, E. L., Resnik, L., Schiefer, M. A., Schmitt, M. S. & Tyler, D. J. Home use of a neural-connected sensory prosthesis provides the functional and psychosocial experience of having a hand again. Sci. Rep. 8 , 9866 (2018).
Weir, R. F., Troyk, P. R., DeMichele, G., Kuiken, T. & Ku, T. Implantable myoelectric sensors (IMES) for upper-extremity prosthesis control—preliminary work. Annu. Int. Conf. IEEE Eng. Med. Biol. Soc. 25 , 1562–1565 (2003).
Merrill, D. R., Lockhart, J., Troyk, P. R., Weir, R. F. & Hankin, D. L. Development of an implantable myoelectric sensor for advanced prosthesis control. Artif. Organs 35 , 249–252 (2011).
Baker, J. J., Scheme, E., Englehart, K., Hutchinson, D. T. & Greger, B. Continuous detection and decoding of dexterous finger flexions with implantable myoelectric sensors. IEEE Trans. Neural Syst. Rehabil. Eng. 18 , 424–432 (2010).
Kristjansson, K. et al. in Converging Clinical and Engineering Research on Neurorehabilitation II (eds Ibáñez, J. et al.) 571–574 (Springer, 2017).
Salminger, S. Long-term implant of intramuscular sensors and nerve transfers for wireless control of robotic arms in above-elbow amputees. Sci. Robot. 4 , eaaw6306 (2019).
Jezernik, S., Grill, W. W. & Sinkjaer, T. Neural network classification of nerve activity recorded in a mixed nerve. Neurol. Res. 23 , 429–434 (2001).
Haugland, M. K. & Sinkjaer, T. Cutaneous whole nerve recordings used for correction of footdrop in hemiplegic man. IEEE Trans. Rehabil. Eng. 3 , 307–317 (1995).
Hoffer, J. & Loeb, G. Implantable electrical and mechanical interfaces with nerve and muscle. Ann. Biomed. Eng. 8 , 351–360 (1980).
Navarro, X. et al. A critical review of interfaces with the peripheral nervous system for the control of neuroprostheses and hybrid bionic systems. J. Peripher. Nerv. Syst. 10 , 229–258 (2005).
Micera, S. et al. Decoding of grasping information from neural signals recorded using peripheral intrafascicular interfaces. J. Neuroeng. Rehabil. 8 , 53 (2011).
Raspopović, S., Capogrosso, M., Navarro, X. & Micera, S. Finite element and biophysics modelling of intraneural transversal electrodes: influence of active site shape. In 2010 Annual International Conference of the IEEE Engineering in Medicine and Biology 1678–1681 (IEEE, 2010).
Kagan, Z. B. et al. Linear methods for reducing EMG contamination in peripheral nerve motor decodes. In 2016 38th Annual International Conference of the IEEE Engineering in Medicine and Biology Society (EMBC) 3422–3425 (IEEE, 2016).
Davis, T. S. et al. Restoring motor control and sensory feedback in people with upper extremity amputations using arrays of 96 microelectrodes implanted in the median and ulnar nerves. J. Neural Eng. 13 , 036001 (2016).
Noce, E. et al. EMG and ENG-envelope pattern recognition for prosthetic hand control. J. Neurosci. Methods 311 , 38–46 (2019).
Petrini, F. M. et al. Microneurography as a tool to develop decoding algorithms for peripheral neuro-controlled hand prostheses. Biomed. Eng. Online 18 , 44 (2019).
Cracchiolo, M. et al. Decoding of grasping tasks from intraneural recordings in trans-radial amputee. J. Neural Eng. 17 , 026034 (2020).
Rossini, P. M. et al. Double nerve intraneural interface implant on a human amputee for robotic hand control. Clin. Neurophysiol. 121 , 777–783 (2010).
Wurth, S. et al. Long-term usability and bio-integration of polyimide-based intra-neural stimulating electrodes. Biomaterials 122 , 114–129 (2017).
Kuiken, T. A. et al. Targeted muscle reinnervation for real-time myoelectric control of multifunction artificial arms. JAMA 301 , 619–628 (2009).
Dumanian, G. A. et al. Targeted reinnervation for transhumeral amputees: current surgical technique and update on results. Plast. Reconstr. Surg. 124 , 863–869 (2009).
Kuiken, T. A., Dumanian, G. A., Lipschutz, R. D., Miller, L. A. & Stubblefield, K. A. The use of targeted muscle reinnervation for improved myoelectric prosthesis control in a bilateral shoulder disarticulation amputee. Prosthet. Orthot. Int. 28 , 245–253 (2004).
Farina, D. et al. Man/machine interface based on the discharge timings of spinal motor neurons after targeted muscle reinnervation. Nat. Biomed. Eng. 1 , 0025 (2017).
Aszmann, O. C. et al. Bionic reconstruction to restore hand function after brachial plexus injury: a case series of three patients. Lancet 385 , 2183–2189 (2015).
Muceli, S. et al. Decoding motor neuron activity from epimysial thin-film electrode recordings following targeted muscle reinnervation. J. Neural Eng. 16 , 016010 (2019).
Bergmeister, K. D. et al. Peripheral nerve transfers induce target muscle hyper-reinnervation and muscle fiber type switch. Sci. Adv. 5 , eaau2956 (2019).
Farina, D. et al. Noninvasive, accurate assessment of the behavior of representative populations of motor units in targeted reinnervated muscles. IEEE Trans. Neural Syst. Rehabil. Eng. 22 , 810–819 (2014).
Kapelner, T. et al. Motor unit characteristics after targeted muscle reinnervation. PLoS ONE 11 , e0149772 (2016).
Kapelner, T. et al. Classification of motor unit activity following targeted muscle reinnervation. In 2015 7th International IEEE/EMBS Conference on Neural Engineering (NER) 652–654 (IEEE, 2015).
Bergmeister, K. D. et al. Broadband prosthetic interfaces: combining nerve transfers and implantable multichannel EMG technology to decode spinal motor neuron activity. Front. Neurosci. 11 , 421 (2017).
Ortiz-Catalan, M. Neuroengineering: deciphering neural drive. Nat. Biomed. Eng. 1 , 0034 (2017).
Chen, C. et al. Prediction of finger kinematics from discharge timings of motor units: implications for intuitive control of myoelectric prostheses. J. Neural Eng. 16 , 026005 (2019).
Urbanchek, M. G. et al. Development of a regenerative peripheral nerve interface for control of a neuroprosthetic limb. BioMed. Res. Int. 2016 , 1–8 (2016).
Frost, C. M. et al. Regenerative peripheral nerve interfaces for real-time, proportional control of a neuroprosthetic hand. J. Neuroeng. Rehabil. 15 , 108 (2018).
Vu, P. P. et al. Closed-loop continuous hand control via chronic recording of regenerative peripheral nerve interfaces. IEEE Trans. Neural Syst. Rehabil. Eng. 26 , 515–526 (2018).
Vu, P. P. et al. A regenerative peripheral nerve interface allows real-time control of an artificial hand in upper limb amputees. Sci. Transl. Med. 12 , eaay2857 (2020).
Collinger, J. L. et al. High-performance neuroprosthetic control by an individual with tetraplegia. Lancet 381 , 557–564 (2013).
Wodlinger, B. et al. Ten-dimensional anthropomorphic arm control in a human brain–machine interface: difficulties, solutions, and limitations. J. Neural Eng. 12 , 016011 (2015).
Courtine, G., Micera, S., DiGiovanna, J. & del R Millán, J. Brain–machine interface: closer to therapeutic reality? Lancet 381 , 515–517 (2013).
Lebedev, M. A. & Nicolelis, M. A. L. Brain–machine interfaces: past, present and future. Trends Neurosci. 29 , 536–546 (2006).
Rohm, M. et al. Hybrid brain–computer interfaces and hybrid neuroprostheses for restoration of upper limb functions in individuals with high-level spinal cord injury. Artif. Intell. Med. 59 , 133–142 (2013).
Ison, M., Vujaklija, I., Whitsell, B., Farina, D. & Artemiadis, P. High-density electromyography and motor skill learning for robust long-term control of a 7-DoF robot arm. IEEE Trans. Neural Syst. Rehabil. Eng. 24 , 424–433 (2016).
Makin, T. R., de Vignemont, F. & Faisal, A. A. Neurocognitive barriers to the embodiment of technology. Nat. Biomed. Eng. 1 , 0014 (2017).
Tyler, D. J. Neural interfaces for somatosensory feedback. Curr. Opin. Neurol. 28 , 574–581 (2015).
Jiang, N., Rehbaum, H., Vujaklija, I., Graimann, B. & Farina, D. Intuitive, online, simultaneous, and proportional myoelectric control over two degrees-of-freedom in upper limb amputees. IEEE Trans. Neural Syst. Rehabil. Eng. 22 , 501–510 (2014).
Amsuess, S. et al. Context-dependent upper limb prosthesis control for natural and robust use. IEEE Trans. Neural Syst. Rehabil. Eng. 24 , 744–753 (2016).
Smith, L. H., Kuiken, T. A. & Hargrove, L. J. Real-time simultaneous myoelectric control by transradial amputees using linear and probability-weighted regression. In 2015 37th Annual International Conference of the IEEE Engineering in Medicine and Biology Society (EMBC) 1119–1123 (IEEE, 2015).
Hahne, J. M., Schweisfurth, M. A., Koppe, M. & Farina, D. Simultaneous control of multiple functions of bionic hand prostheses: performance and robustness in end users. Sci. Robot. 3 , eaat3630 (2018).
Vujaklija, I. et al. Online mapping of EMG signals into kinematics by autoencoding. J. Neuroeng. Rehabil. 15 , 21 (2018).
Hahne, J. M., Markovic, M. & Farina, D. User adaptation in myoelectric man-machine interfaces. Sci. Rep. 7 , 4437 (2017).
Sartori, M., Llyod, D. G. & Farina, D. Neural data-driven musculoskeletal modeling for personalized neurorehabilitation technologies. IEEE Trans. Biomed. Eng. 63 , 879–893 (2016).
Sartori, M., Farina, D. & Lloyd, D. G. Hybrid neuromusculoskeletal modeling to best track joint moments using a balance between muscle excitations derived from electromyograms and optimization. J. Biomech. 47 , 3613–3621 (2014).
Durandau, G., Farina, D. & Sartori, M. Robust real-time musculoskeletal modeling driven by electromyograms. IEEE Trans. Biomed. Eng. 65 , 556–564 (2018).
Crouch, D. L. & Huang, H. Lumped-parameter electromyogram-driven musculoskeletal hand model: a potential platform for real-time prosthesis control. J. Biomech. 49 , 3901–3907 (2016).
Crouch, D. L. & Huang, H. Musculoskeletal model-based control interface mimics physiologic hand dynamics during path tracing task. J. Neural Eng. 14 , 036008 (2017).
Sartori, M., Durandau, G., Došen, S. & Farina, D. Robust simultaneous myoelectric control of multiple degrees of freedom in wrist-hand prostheses by real-time neuromusculoskeletal modeling. J. Neural Eng. 15 , 066026 (2018).
Sartori, M., Reggiani, M., Farina, D. & Lloyd, D. G. EMG-driven forward-dynamic estimation of muscle force and joint moment about multiple degrees of freedom in the human lower extremity. PLoS ONE 7 , e52618 (2012).
Sartori, M., van de Riet, J. & Farina, D. Estimation of phantom arm mechanics about four degrees of freedom after targeted muscle reinnervation. IEEE Trans. Med. Robot. Bionics 1 , 58–64 (2019).
Young, A. J., Simon, A. M. & Hargrove, L. J. A training method for locomotion mode prediction using powered lower limb prostheses. IEEE Trans. Neural Syst. Rehabil. Eng. 22 , 671–677 (2014).
Simon, A. M. et al. Configuring a powered knee and ankle prosthesis for transfemoral amputees within five specific ambulation modes. PLoS ONE 9 , e99387 (2014).
Huang, H., Kuiken, T. A. & Lipschutz, R. D. A strategy for identifying locomotion modes using surface electromyography. IEEE Trans. Biomed. Eng. 56 , 65–73 (2009).
Wang, J., Kannape, O. A. & Herr, H. M. Proportional EMG control of ankle plantar flexion in a powered transtibial prosthesis. In 2013 IEEE 13th International Conference on Rehabilitation Robotics (ICORR) 1–5 (IEEE, 2013).
Spanias, J. A., Perreault, E. J. & Hargrove, L. J. Detection of and compensation for EMG disturbances for powered lower limb prosthesis control. IEEE Trans. Neural Syst. Rehabil. Eng. 24 , 226–234 (2016).
Scheme, E., Fougner, A., Stavdahl, Ø., Chan, A. D. C. & Englehart, K. Examining the adverse effects of limb position on pattern recognition based myoelectric control. In 2010 Annual International Conference of the IEEE Engineering in Medicine and Biology 6337–6340 (IEEE, 2010).
Scheme, E. J., Englehart, K. B. & Hudgins, B. S. Selective classification for improved robustness of myoelectric control under nonideal conditions. IEEE Trans. Biomed. Eng. 58 , 1698–1705 (2011).
Sensinger, J. W., Lock, B. A. & Kuiken, T. A. Adaptive pattern recognition of myoelectric signals: exploration of conceptual framework and practical algorithms. IEEE Trans. Neural Syst. Rehabil. Eng. 17 , 270–278 (2009).
Zhang, F. & Huang, H. Source selection for real-time user intent recognition toward volitional control of artificial legs. IEEE J. Biomed. Health Inform. 17 , 907–914 (2013).
Article PubMed Central Google Scholar
Hahne, J. M., Dahne, S., Hwang, H.-J., Muller, K.-R. & Parra, L. C. Concurrent adaptation of human and machine improves simultaneous and proportional myoelectric control. IEEE Trans. Neural Syst. Rehabil. Eng. 23 , 618–627 (2015).
Yeung, D., Farina, D. & Vujaklija, I. Directional forgetting for stable co-adaptation in myoelectric control. Sensors 19 , 2203 (2019).
Edwards, A. L. et al. Application of real-time machine learning to myoelectric prosthesis control: a case series in adaptive switching. Prosthet. Orthot. Int. 40 , 573–581 (2016).
Spanias, J. A., Simon, A. M., Perreault, E. J. & Hargrove, L. J. Preliminary results for an adaptive pattern recognition system for novel users using a powered lower limb prosthesis. In 2016 38th Annual International Conference of the IEEE Engineering in Medicine and Biology Society (EMBC) 5083–5086 (IEEE, 2016).
Du, L., Zhang, F., He, H. & Huang, H. Improving the performance of a neural-machine interface for prosthetic legs using adaptive pattern classifiers. In Proc. Annual International Conference of the IEEE Engineering in Medicine and Biology Society (EMBC) 1571–1574 (IEEE, 2013).
Zhuang, K. Z. et al. Shared human–robot proportional control of a dexterous myoelectric prosthesis. Nat. Mach. Intell. 1 , 400–411 (2019).
Volkmar, R., Dosen, S., Gonzalez-Vargas, J., Baum, M. & Markovic, M. Improving bimanual interaction with a prosthesis using semi-autonomous control. J. Neuroeng. Rehabil. 16 , 140 (2019).
Bensmaia, S. J., Tyler, D. J. & Micera, S. Restoration of sensory information via bionic hands. Nat. Biomed. Eng. https://doi.org/10.1038/s41551-020-00630-8 (2020).
Berniker, M. & Kording, K. Bayesian approaches to sensory integration for motor control. Wiley Interdiscip. Rev. Cogn. Sci. 2 , 419–428 (2011).
Witteveen, H. J., Rietman, H. S. & Veltink, P. H. Vibrotactile grasping force and hand aperture feedback for myoelectric forearm prosthesis users. Prosthet. Orthot. Int. 39 , 204–212 (2015).
Dosen, S., Ninu, A., Yakimovich, T., Dietl, H. & Farina, D. A novel method to generate amplitude-frequency modulated vibrotactile stimulation. IEEE Trans. Haptics 9 , 3–12 (2016).
Antfolk, C., Balkenius, C., Lundborg, G., Rosen, B. & Sebelius, F. A tactile display system for hand prostheses to discriminate pressure and individual finger localization. J. Med. Biol. Eng. 30 , 355–359 (2010).
Antfolk, C. et al. Artificial redirection of sensation from prosthetic fingers to the phantom hand map on transradial amputees: vibrotactile versus mechanotactile sensory feedback. IEEE Trans. Neural Syst. Rehabil. Eng. 21 , 112–120 (2013).
Bark, K., Wheeler, J., Lee, G., Savall, J. & Cutkosky, M. A wearable skin stretch device for haptic feedback. In World Haptics 2009—3rd Joint EuroHaptics Conference and Symposium on Haptic Interfaces for Virtual Environment and Teleoperator Systems 464–469 (IEEE, 2009).
Wheeler, J., Bark, K., Savall, J. & Cutkosky, M. Investigation of rotational skin stretch for proprioceptive feedback with application to myoelectric systems. IEEE Trans. Neural Syst. Rehabil. Eng. 18 , 58–66 (2010).
Patterson, P. E. & Katz, J. A. Design and evaluation of a sensory feedback system that provides grasping pressure in a myoelectric hand. J. Rehabil. Res. Dev. 29 , 1–8 (1992).
Štrbac, M. et al. Integrated and flexible multichannel interface for electrotactile stimulation. J. Neural Eng. 13 , 046014 (2016).
Patel, G. K., Dosen, S., Castellini, C. & Farina, D. Multichannel electrotactile feedback for simultaneous and proportional myoelectric control. J. Neural Eng. 13 , 056015 (2016).
Scott, R. N., Brittain, R. H., Caldwell, R. R., Cameron, A. B. & Dunfield, V. A. Sensory-feedback system compatible with myoelectric control. Med. Biol. Eng. Comput. 18 , 65–69 (1980).
D’Anna, E. et al. A somatotopic bidirectional hand prosthesis with transcutaneous electrical nerve stimulation based sensory feedback. Sci. Rep. 7 , 10930 (2017).
Li, M. et al. Discrimination and recognition of phantom finger sensation through transcutaneous electrical nerve stimulation. Front. Neurosci. 12 , 283 (2018).
Vargas, L. et al. Object stiffness recognition using haptic feedback delivered through transcutaneous proximal nerve stimulation. J. Neural Eng. 17 , 016002 (2019).
Zollo, L. et al. Restoring tactile sensations via neural interfaces for real-time force-and-slippage closed-loop control of bionic hands. Sci. Robot. 4 , eaau9924 (2019).
Jorgovanovic, N., Dosen, S., Djozic, D. J., Krajoski, G. & Farina, D. Virtual grasping: closed-loop force control using electrotactile feedback. Comput. Math. Methods Med . 2014 , 120357 (2014).
Saunders, I. & Vijayakumar, S. The role of feed-forward and feedback processes for closed-loop prosthesis control. J. Neuroeng. Rehabil. 8 , 60 (2011).
Schweisfurth, M. A. et al. Electrotactile EMG feedback improves the control of prosthesis grasping force. J. Neural Eng. 13 , 056010 (2016).
Marasco, P. D., Schultz, A. E. & Kuiken, T. A. Sensory capacity of reinnervated skin after redirection of amputated upper limb nerves to the chest. Brain 132 , 1441–1448 (2009).
Kuiken, T. A. et al. Targeted reinnervation for enhanced prosthetic arm function in a woman with a proximal amputation: a case study. Lancet 369 , 371–380 (2007).
Marasco, P. D., Kim, K., Colgate, J. E., Peshkin, M. A. & Kuiken, T. A. Robotic touch shifts perception of embodiment to a prosthesis in targeted reinnervation amputees. Brain 134 , 747–758 (2011).
Srinivasan, S. S. & Herr, H. M. A cutaneous mechanoneural interface for neuroprosthetic feedback. Nat. Biomed. Eng . https://doi.org/10.1038/s41551-020-00669-7 (2021).
Čvančara, P. et al. Stability of flexible thin-film metallization stimulation electrodes: analysis of explants after first-in-human study and improvement of in vivo performance. J. Neural Eng. 17 , 046006 (2020).
Raspopovic, S. et al. Restoring natural sensory feedback in real-time bidirectional hand prostheses. Sci. Transl. Med. 6 , 222ra19 (2014).
Tan, D. W. et al. A neural interface provides long-term stable natural touch perception. Sci. Transl. Med. 6 , 257ra138 (2014).
Mastinu, E. et al. Neural feedback strategies to improve grasping coordination in neuromusculoskeletal prostheses. Sci. Rep. 10 , 11793 (2020).
Oddo, C. M. et al. Intraneural stimulation elicits discrimination of textural features by artificial fingertip in intact and amputee humans. eLife 5 , 167–174 (2016).
Petrini, F. M. et al. Six-month assessment of a hand prosthesis with intraneural tactile feedback. Ann. Neurol. 85 , 137–154 (2018).
Valle, G. et al. Biomimetic intraneural sensory feedback enhances sensation naturalness, tactile sensitivity, and manual dexterity in a bidirectional prosthesis. Neuron 100 , 37–45.e7 (2018).
Risso, G. et al. Optimal integration of intraneural somatosensory feedback with visual information: a single-case study. Sci. Rep. 9 , 7916 (2019).
D’Anna, E. et al. A closed-loop hand prosthesis with simultaneous intraneural tactile and position feedback. Sci. Robot. 4 , eaau8892 (2019).
Petrini, F. M. et al. Sensory feedback restoration in leg amputees improves walking speed, metabolic cost and phantom pain. Nat. Med. 25 , 1356–1363 (2019).
Chandrasekaran, S. et al. Sensory restoration by epidural stimulation of the lateral spinal cord in upper-limb amputees. eLife 9 , e54349 (2020).
Klaes, C. et al. A cognitive neuroprosthetic that uses cortical stimulation for somatosensory feedback. J. Neural Eng. 11 , 056024 (2014).
May, T. et al. Detection of optogenetic stimulation in somatosensory cortex by non-human primates—towards artificial tactile sensation. PLoS ONE 9 , e114529 (2014).
Anderson, H. E. & Weir, R. F. ff. On the development of optical peripheral nerve interfaces. Neural Regen. Res. 14 , 425–436 (2019).
Fontaine, A. K. et al. Optical vagus nerve modulation of heart and respiration via heart-injected retrograde AAV. Sci. Rep. 11 , 3664 (2021).
Fontaine, A. K. et al. Optogenetic stimulation of cholinergic fibers for the modulation of insulin and glycemia. Sci. Rep. 11 , 3670 (2021).
Fontaine, A. K., Gibson, E. A., Caldwell, J. H. & Weir, R. F. Optical read-out of neural activity in mammalian peripheral axons: calcium signaling at nodes of Ranvier. Sci. Rep. 7 , 4744 (2017).
De Nunzio, A. M. et al. Tactile feedback is an effective instrument for the training of grasping with a prosthesis at low- and medium-force levels. Exp. Brain Res. 235 , 2457–2559 (2017).
Strbac, M. et al. Short- and long-term learning of feedforward control of a myoelectric prosthesis with sensory feedback by amputees. IEEE Trans. Neural Syst. Rehabil. Eng. 25 , 2133–2145 (2017).
Mulvey, M. R., Fawkner, H. J., Radford, H. E. & Johnson, M. I. Perceptual embodiment of prosthetic limbs by transcutaneous electrical nerve stimulation. Neuromodulation 15 , 42–47 (2012).
Johnson, S. S. & Mansfield, E. Prosthetic training. Phys. Med. Rehabil. Clin. N. Am. 25 , 133–151 (2014).
Wheaton, L. A. Neurorehabilitation in upper limb amputation: understanding how neurophysiological changes can affect functional rehabilitation. J. Neuroeng. Rehabil. 14 , 41 (2017).
Soyer, K., Ünver, B., Tamer, S. & Ülger, Ö. G. The importance of rehabilitation concerning upper extremity amputees: a systematic review. Pakistan J. Med. Sci. 32 , 1312–1319 (2016).
Roche, A. D. et al. A structured rehabilitation protocol for improved multifunctional prosthetic control: a case study. J. Vis. Exp. 105 , e52968 (2015).
Dise-Lewis, J. E. in Comprehensive Management of the Upper-Limb Amputee (eds Atkins, D. J. & Meier, R. H.) 165–172 (Springer, 1989).
Gallagher, P. & MacLachlan, M. Psychological adjustment and coping in adults with prosthetic limbs. Behav. Med. 25 , 117–124 (1999).
Hruby, L. A., Pittermann, A., Sturma, A. & Aszmann, O. C. The Vienna psychosocial assessment procedure for bionic reconstruction in patients with global brachial plexus injuries. PLoS ONE 13 , e0189592 (2018).
Sturma, A., Hruby, L. A., Prahm, C., Mayer, J. A. & Aszmann, O. C. Rehabilitation of upper extremity nerve injuries using surface EMG biofeedback: protocols for clinical application. Front. Neurosci. 12 , 906 (2018).
Vujaklija, I. et al. Translating research on myoelectric control into clinics—are the performance assessment methods adequate? Front. Neurorobot. 11 , 7 (2017).
Ortiz-Catalan, M., Rouhani, F., Branemark, R. & Hakansson, B. Offline accuracy: a potentially misleading metric in myoelectric pattern recognition for prosthetic control. In 2015 37th Annual International Conference of the IEEE Engineering in Medicine and Biology Society (EMBC) 1140–1143 (IEEE, 2015).
Jarvis, H. L. et al. Temporal spatial and metabolic measures of walking in highly functional individuals with lower limb amputations. Arch. Phys. Med. Rehabil. 98 , 1389–1399 (2017).
Smurr, L. M., Gulick, K., Yancosek, K. & Ganz, O. Managing the upper extremity amputee: a protocol for success. J. Hand Ther. 21 , 160–176 (2008).
Sturma, A. et al. Rehabilitation of high upper limb amputees after targeted muscle reinnervation. J. Hand Ther . https://doi.org/10.1016/j.jht.2020.10.002 (2020).
Prahm, C., Vujaklija, I., Kayali, F., Purgathofer, P. & Aszmann, O. C. Game-based rehabilitation for myoelectric prosthesis control. JMIR Serious Games 5 , e3 (2017).
Anderson, F. & Bischof, W. F. Augmented reality improves myoelectric prosthesis training. Int. J. Disabil. Hum. Dev. 13 , 349–354 (2014).
Prahm, C., Kayali, F., Sturma, A. & Aszmann, O. PlayBionic: game-based interventions to encourage patient engagement and performance in prosthetic motor rehabilitation. PM&R 10 , 1252–1260 (2018).
Tillander, J., Hagberg, K., Hagberg, L. & Brånemark, R. Osseointegrated titanium implants for limb prostheses attachments: infectious complications. Clin. Orthop. Relat. Res. 468 , 2781–2788 (2010).
Delgado-Martinez, I. et al. Fascicular nerve stimulation and recording using a novel double-aisle regenerative electrode. J. Neural Eng. 14 , 046003 (2017).
Tan, D. W., Schiefer, M. A., Keith, M. W., Anderson, J. R. & Tyler, D. J. Stability and selectivity of a chronic, multi-contact cuff electrode for sensory stimulation in human amputees. J. Neural Eng. 12 , 026002 (2015).
Micera, S., Caleo, M., Chisari, C., Hummel, F. C. & Pedrocchi, A. Advanced neurotechnologies for the restoration of motor function. Neuron 105 , 604–620 (2020).
Delianides, C., Tyler, D., Pinault, G., Ansari, R. & Triolo, R. Implanted high density cuff electrodes functionally activate human tibial and peroneal motor units without chronic detriment to peripheral nerve health. Neuromodulation 23 , 754–762 (2020).
Paggi, V., Akoussi, O., Micera, S. & Lacour, P. S. Compliant peripheral nerve interfaces. J. Neural Eng. 18 , 031001 (2021).
Srinivasan, S. S. et al. On prosthetic control: a regenerative agonist-antagonist myoneural interface. Sci. Robot. 2 , eaan2971 (2017).
Horch, K., Meek, S., Taylor, T. G. & Hutchinson, D. T. Object discrimination with an artificial hand using electrical stimulation of peripheral tactile and proprioceptive pathways with intrafascicular electrodes. IEEE Trans. Neural Syst. Rehabil. Eng. 19 , 483–489 (2011).
Schiefer, M. A., Graczyk, E. L., Sidik, S. M., Tan, D. W. & Tyler, D. J. Artificial tactile and proprioceptive feedback improves performance and confidence on object identification tasks. PLoS ONE 13 , e0207659 (2018).
Fernández, A., Isusi, I. & Gómez, M. Factors conditioning the return to work of upper limb amputees in Asturias, Spain. Prosthet. Orthot. Int. 24 , 143–147 (2000).
Burger, H. & Marinček, Č. Return to work after lower limb amputation. Disabil. Rehabil. 29 , 1323–1329 (2007).
Stieglitz, T. Of man and mice: translational research in neurotechnology. Neuron 105 , 12–15 (2020).
van der Sluis, C. K. & Bongers, R. M. TIPS for scaling up research in upper limb prosthetics. Prosthesis 2 , 340–351 (2020).
Hickey, G., Richards, T. & Sheehy, J. Co-production from proposal to paper. Nature 562 , 29–31 (2018).
Vasudevan, S., Patel, K. & Welle, C. Rodent model for assessing the long term safety and performance of peripheral nerve recording electrodes. J. Neural Eng. 14 , 016008 (2017).
Sartoretto, S. C. et al. Sheep as an experimental model for biomaterial implant evaluation. Acta Ortop. Bras. 24 , 262–266 (2016).
Boretius, T. et al. A transverse intrafascicular multichannel electrode (TIME) to interface with the peripheral nerve. Biosens. Bioelectron. 26 , 62–69 (2010).
Tyler, D. J. & Durand, D. M. Chronic response of the rat sciatic nerve to the flat interface nerve electrode. Ann. Biomed. Eng. 31 , 633–642 (2003).
Download references
Acknowledgements
We were supported by the Academy of Finland (I.V.), Austrian Federal Ministry of Science (A.S. and O.C.A.), Bertarelli Foundation (S.M.), the European Union (A.S., D.F., K.-P.H., O.C.A., R.B. and S.M.), the European Research Council (A.S., D.F. and O.C.A.), German Federal Ministry of Education and Research BMBF (K.-P.H. and T.S.), the German National Research Foundation (T.S.), the Royal British Legion (A.M.J.B.), the Swedish Innovation Agency (VINNOVA) (R.B.), the Swedish Research Council (R.B.), the Swiss National Competence Center in Research (NCCR) in Robotics (S.M.), US Department of Defense (R.B. and H.H.), US Department of Veterans Affairs (D.T.), US Department of Veterans Affairs Rehabilitation Research and Development Service (R.F.ff.W.), US National Institute on Disability, Independent Living and Rehabilitation Research (H.H. and T.K.), US National Institutes of Health (D.T., H.H., L.J.H. and R.F.ff.W.), US National Institute on Neurological Disorders and Stroke (R.F.ff.W.), US National Institute on Bioimaging and Bioengineering (R.F.ff.W.) and US National Science Foundation (H.H.).
Author information
Authors and affiliations.
Department of Bioengineering, Imperial College London, London, UK
Dario Farina, Anthony M. J. Bull & Agnes Sturma
Department of Electrical Engineering and Automation, Aalto University, Espoo, Finland
Ivan Vujaklija
Center for Extreme Bionics, Biomechatronics Group, MIT Media Lab, Massachusetts Institute of Technology, Cambridge, MA, USA
Rickard Brånemark
Department of Orthopaedics, Institute of Clinical Sciences, Sahlgrenska Academy, University of Gothenburg, Sahlgrenska University Hospital, Gothenburg, Sweden
Ottobock Products SE & Co. KGaA, Vienna, Austria
Ottobock SE & Co. KGaA, Duderstadt, Germany
Bernhard Graimann
Center for Bionic Medicine, Shirley Ryan AbilityLab, Chicago, IL, USA
Levi J. Hargrove & Todd Kuiken
Department of Physical Medicine & Rehabilitation, Northwestern University, Chicago, IL, USA
Department of Biomedical Engineering, Northwestern University, Chicago, IL, USA
Department of Medical Engineering & Neuroprosthetics, Fraunhofer-Institut für Biomedizinische Technik, Sulzbach, Germany
Klaus-Peter Hoffmann
NCSU/UNC Joint Department of Biomedical Engineering, North Carolina State University, Raleigh, NC, USA
He (Helen) Huang
University of North Carolina at Chapel Hill, Chapel Hill, NC, USA
Department of Research and Development, Össur Iceland, Reykjavík, Iceland
Thorvaldur Ingvarsson & Kristleifur Kristjánsson
Faculty of Medicine, University of Iceland, Reykjavík, Iceland
Thorvaldur Ingvarsson
School of Engineering and Natural Sciences, University of Iceland, Reykjavík, Iceland
Hilmar Bragi Janusson
The Biorobotics Institute and Department of Excellence in Robotics and AI, Scuola Superiore Sant’Anna, Pontedera, Italy
Silvestro Micera
Department of Excellence in Robotics and AI, Scuola Superiore Sant’Anna, Pontedera, Italy
Bertarelli Foundation Chair in Translational NeuroEngineering, Center for Neuroprosthetics and Institute of Bioengineering, School of Engineering, Ecole Polytechnique Federale de Lausanne, Lausanne, Switzerland
Laboratory for Biomedical Microtechnology, Department of Microsystems Engineering-IMTEK, BrainLinks-BrainTools Center and Bernstein Center Freiburg, University of Freiburg, Freiburg, Germany
Thomas Stieglitz
Clinical Laboratory for Bionic Extremity Reconstruction, Department of Plastic and Reconstructive Surgery, Medical University of Vienna, Vienna, Austria
Agnes Sturma & Oskar C. Aszmann
Case School of Engineering, Case Western Reserve University, Cleveland, OH, USA
Dustin Tyler
Louis Stokes Veterans Affairs Medical Centre, Cleveland, OH, USA
Biomechatronics Development Laboratory, Bioengineering Department, University of Colorado Denver and VA Eastern Colorado Healthcare System, Aurora, CO, USA
Richard F. ff. Weir
You can also search for this author in PubMed Google Scholar
Contributions
D.F. and O.C.A. conceived the project, and D.F., I.V., A.S. and O.C.A. edited the manuscript. All authors contributed to writing and revising the manuscript, and approved the final version.
Corresponding author
Correspondence to Dario Farina .
Ethics declarations
Competing interests.
L.J.H. and T.K. have a financial interest in Coapt LLC ( https://www.coaptengineering.com ). S.M. is a co-founder of Sensars Neuroprosthetics ( https://www.sensars.com ). T.S. is a co-founder and scientific advisor of CorTec GmbH ( https://www.cortec-neuro.com ) and neuroloop GmbH ( https://www.neuroloop.de ). R.F.ff.W. is a co-founder and president of Point Designs Llc ( https://www.pointdesignsllc.com ). H.D. and B.G. are scientific managers at Ottobock SE & Co. KGaA. T.I. and K.K. are scientific officers at Össur Iceland. R.B. is the founder and chairman of Integrum AB. A.M.J.B. is co-founder and director of Biomex Ltd.
Additional information
Publisher’s note Springer Nature remains neutral with regard to jurisdictional claims in published maps and institutional affiliations.
Rights and permissions
Reprints and permissions
About this article
Cite this article.
Farina, D., Vujaklija, I., Brånemark, R. et al. Toward higher-performance bionic limbs for wider clinical use. Nat. Biomed. Eng 7 , 473–485 (2023). https://doi.org/10.1038/s41551-021-00732-x
Download citation
Received : 27 February 2019
Accepted : 01 April 2021
Published : 31 May 2021
Issue Date : April 2023
DOI : https://doi.org/10.1038/s41551-021-00732-x
Share this article
Anyone you share the following link with will be able to read this content:
Sorry, a shareable link is not currently available for this article.
Provided by the Springer Nature SharedIt content-sharing initiative
This article is cited by
Shaping high-performance wearable robots for human motor and sensory reconstruction and enhancement.
- Haisheng Xia
- Yuchong Zhang
Nature Communications (2024)
Biomimetic versus arbitrary motor control strategies for bionic hand skill learning
- Hunter R. Schone
- Malcolm Udeozor
- Chris I. Baker
Nature Human Behaviour (2024)
Automated calibration of somatosensory stimulation using reinforcement learning
- Luigi Borda
- Noemi Gozzi
- Stanisa Raspopovic
Journal of NeuroEngineering and Rehabilitation (2023)
The SoftHand Pro platform: a flexible prosthesis with a user-centered approach
- Patricia Capsi-Morales
- Cristina Piazza
- Manuel G. Catalano
A myoelectric digital twin for fast and realistic modelling in deep learning
- Kostiantyn Maksymenko
- Alexander Kenneth Clarke
- Dario Farina
Nature Communications (2023)
Quick links
- Explore articles by subject
- Guide to authors
- Editorial policies
Sign up for the Nature Briefing: Translational Research newsletter — top stories in biotechnology, drug discovery and pharma.

Differences in myoelectric and body-powered upper-limb prostheses: Systematic literature review
Affiliation.
- 1 Department of Mechanical Engineering, University of South Florida, Tampa, FL;
- PMID: 26230500
- DOI: 10.1682/JRRD.2014.08.0192
The choice of a myoelectric or body-powered upper-limb prosthesis can be determined using factors including control, function, feedback, cosmesis, and rejection. Although body-powered and myoelectric control strategies offer unique functions, many prosthesis users must choose one. A systematic review was conducted to determine differences between myoelectric and body-powered prostheses to inform evidence-based clinical practice regarding prescription of these devices and training of users. A search of 9 databases identified 462 unique publications. Ultimately, 31 of them were included and 11 empirical evidence statements were developed. Conflicting evidence has been found in terms of the relative functional performance of body-powered and myoelectric prostheses. Body-powered prostheses have been shown to have advantages in durability, training time, frequency of adjustment, maintenance, and feedback; however, they could still benefit from improvements of control. Myoelectric prostheses have been shown to improve cosmesis and phantom-limb pain and are more accepted for light=intensity work. Currently, evidence is insufficient to conclude that either system provides a significant general advantage. Prosthetic selection should be based on a patient's individual needs and include personal preferences, prosthetic experience, and functional needs. This work demonstrates that there is a lack of empirical evidence regarding functional differences in upper-limb prostheses.
Keywords: amputation; artificial limb; control; cosmesis; external power; function; prosthesis; rehabilitation; transhumeral; transradial.
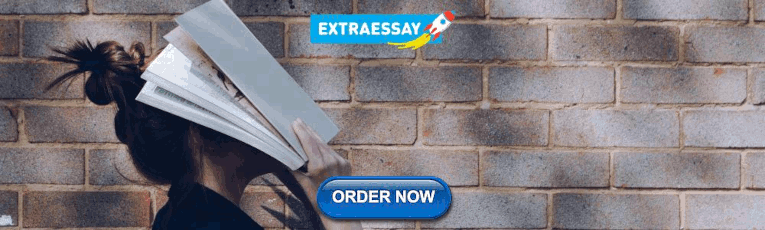
Publication types
- Research Support, Non-U.S. Gov't
- Systematic Review
- Amputees / rehabilitation*
- Arm / surgery*
- Artificial Limbs*
- Biomedical Engineering / methods*
- Electromyography
- Prosthesis Design
Click through the PLOS taxonomy to find articles in your field.
For more information about PLOS Subject Areas, click here .
Loading metrics
Open Access
Peer-reviewed
Research Article
What do users and their aiding professionals want from future devices in upper limb prosthetics? A focus group study
Roles Writing – original draft
¶ ‡ AKE and FR share first authorship on this work.
Affiliation Laboratory for Biomechanics and Biomaterials, Department of Orthopedic Surgery, Hannover Medical School, Hannover, Germany

Roles Data curation, Investigation, Methodology, Writing – original draft, Writing – review & editing
Roles Supervision
Affiliation Department of Orthopedic Surgery, Hannover Medical School, Hannover, Germany
Roles Writing – review & editing
Roles Funding acquisition
Roles Conceptualization, Data curation, Funding acquisition, Writing – review & editing
* E-mail: [email protected]
- Ann-Kathrin Einfeldt,
- Franziska Rebmann,
- Dawei Yao,
- Christina Stukenborg-Colsmann,
- Christof Hurschler,
- Henning Windhagen,
- Eike Jakubowitz
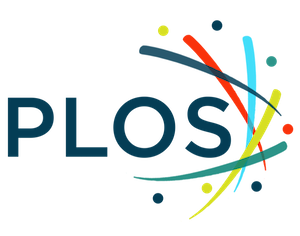
- Published: December 29, 2023
- https://doi.org/10.1371/journal.pone.0295516
- Reader Comments
High rejection rates of upper limb prosthetics indicate that current prosthetic devices only partially meet user demands. This study therefore investigated the benefits and challenges with current prostheses, associated services and potential areas for improvement from the perspective of upper limb prosthesis users and various professionals working in the field of upper limb and hand prosthetics.
Methods and findings
Seven different focus group discussions were conducted with 32 participants. Participants were grouped by prosthesis type, if they were prosthesis users, or professionals. All focus group discussions were transcribed verbatim, and a summarizing content analysis was performed. Three main topic areas to be addressed emerged from the interviews: 1. a properly functioning prosthesis, 2. the infrastructure, and 3. users’ psychological and physical prerequisites. The interaction between a well-functioning prosthesis and a well-developed infrastructure was shown to be important for successful use.
Conclusions
Our study raises many of the same issues that have been reported in previous qualitative studies, dating back over several decades. This study underlines the need to include users and professionals in the future development of prosthetic devices.
Citation: Einfeldt A-K, Rebmann F, Yao D, Stukenborg-Colsmann C, Hurschler C, Windhagen H, et al. (2023) What do users and their aiding professionals want from future devices in upper limb prosthetics? A focus group study. PLoS ONE 18(12): e0295516. https://doi.org/10.1371/journal.pone.0295516
Editor: Noman Naseer, Air University, PAKISTAN
Received: March 16, 2023; Accepted: November 22, 2023; Published: December 29, 2023
Copyright: © 2023 Einfeldt et al. This is an open access article distributed under the terms of the Creative Commons Attribution License , which permits unrestricted use, distribution, and reproduction in any medium, provided the original author and source are credited.
Data Availability: All relevant data are within the manuscript. The anonymized transcripts in German language will be uploaded in the Supporting Information files. The original video files from the focus group discussions cannot be shared because the data contains identifying patient information.
Funding: This study received funding from the European Union’s Horizon 2020 Research and Innovation Programme under Grant Agreement No. 688857 called “SoftPro”. The funders had no role in study design, data collection and analysis, decision to publish, or preparation of the manuscript.
Competing interests: The authors have declared that no competing interests exist.
Introduction
According to the German Federal Statistical Office, 225 amputations and exarticulations of the upper extremity were performed in Germany in 2019 [ 1 ]. Cases of amputations of the hand were significantly higher with 5650 patients affected [ 1 ]. In 2014, these numbers were 152 and 4047, respectively, which means there is an increasing trend. Even though various treatment options are available in upper limb prosthetics, high rejection rates indicate that current prosthetic devices meet the needs of users only partially or not at all [ 2 , 3 ]. Therefore, users and professionals should be included in the research and development process for new prostheses. Many quantitative studies examining the opinions of prosthesis users (PUs) towards their prosthesis and their use of it have been performed [ 4 , 5 ]. Qualitative studies have the potential to highlight patients’ subjective needs and perspectives [ 6 ]. Detailed research and evaluations in qualitative studies show that these studies investigate users’ and experts’ opinions with limitations regarding specific requirements for prosthesis use or care [ 2 , 7 – 10 ]. Therefore, this study aimed to investigate, in an open-ended manner, which characteristics and properties of myoelectric prostheses could be advantageous from the perspective of upper limb prosthesis users and professionals working in upper limb and hand prosthetics. The results will be used to facilitate future developments in this field to support the design of appropriate products and better meet user needs.
The study is part of the SoftPro project (Synergy-based Opensource Foundations and Technologies for Prosthetics and Rehabilitation) . Its purpose is to integrate patient needs and expert opinions in prosthetic care into the implementation process of transforming the PISA/IIT SoftHand 2 [ 11 ], initially developed as an end effector in robotics, into a version for use in upper limb prosthetics (SoftHand Pro).
Consequently, this study addresses the following research questions about upper limb prostheses:
- What are the most important requirements for users and professionals?
- Have technical innovations in recent years changed the requirements of users and professionals?
- Which unfulfilled requirements of past prosthetic devices can be addressed with future prosthetic devices?
Seven focus group (FG) discussions were conducted between 26.10.2017 and 05.12.2017 with 32 participants from four German states (Baden-Württemberg, Berlin, Lower Saxony, and North Rhine-Westphalia). All methods were reviewed and approved by the local ethics committee of Hannover Medical School (No: 3531).
Participants
Participants included PUs, orthopedic technicians (OTs), and therapists (THs), including physiotherapists (PHTs) as well as occupational therapists (OCTs) recruited using predefined inclusion and exclusion criteria ( Table 1 ). PUs were recruited through different medical care providers in Lower Saxony. OTs were recruited by contacting orthotics and prosthetics providers in Baden-Württemberg and Hannover. PHTs and OCTs were recruited via a German directory, including specialized therapists for upper limb amputees and their prosthetic care. According to Pollock and Böhm [ 12 ], each focus group should consist of at least three to a maximum of six participants. Participants were not mixed but instead assigned according to specific target groups (PUs and different professionals) to enable a holistic processing of the research questions and achieve FGs that were as consistent as possible. A more differentiated focus on the prosthetic hand was realized by further consolidating the PU group into subgroups based on the control aspects and kinematics of their prosthetic end effectors ( Fig 1 ).
- PPT PowerPoint slide
- PNG larger image
- TIFF original image
https://doi.org/10.1371/journal.pone.0295516.g001
https://doi.org/10.1371/journal.pone.0295516.t001
Data collection and analysis
First, a guideline for conducting interviews based on the recommendations by Helfferich [ 13 ] was created, and seven to nine key questions were drafted for each FG. This approach resulted in three group-specific guidelines (PUs, OTs, and THs; Tables 2 & 3 ). These were not intended to shape the interview as a strict list of questions, but rather to help guide the conversation along topics of interest. They were discussed amongst various research groups with clinical, technical, and bioethical expertise and piloted by two amputation surgery physicians, but no revisions were raised.
https://doi.org/10.1371/journal.pone.0295516.t002
https://doi.org/10.1371/journal.pone.0295516.t003
Coherency was ensured by a single moderator (MD candidate, female), who performed all FGs and was responsible for examining the collected data. A passive assistant operated the audio recordings using two microphones and documented participants’ non-verbal communication (body language and mimic expressions). The moderator ensured open-ended questions and the spontaneous addition of new topics as they arose. All FGs were held in German. After each FG, PUs were asked to fill out a questionnaire concerning their sociodemographic and prosthetic data. The questionnaire also collected data regarding prosthesis using times and satisfaction with the prosthesis on a ten point rating scale. Based on the transcription rules according to Bohnsack [ 14 ], all FGs were transcribed verbatim directly afterwards using a guide and the software f5transkript (Vers. 7, Dr. Dresing und Pehl GmbH, Marburg, Germany). Following Mayring and Fenzl [ 15 ], the transcripts were evaluated using summarizing content analysis, a method to summarize content through a process of systematic reductions. Individual sections of the transcription were first paraphrased and then generalized, whereas text components without relevant content were deleted and content-bearing components were brought into a uniform grammatical form. This allowed different categories to be defined and the frequency of their occurrence determined their relevance. The categories where not defined beforehand, which means an inductive category system was used. The category system was worked out using the software MAXQDA Analytics Pro (Vers. 2018, Verbi GmbH, Berlin, Germany).
Nineteen PUs ( Table 4 ), nine OTs, and four THs ( Table 5 ) were enrolled in this study. Of the PUs, 83% were male and 61% working. The ratio of acquired to congenital amputations is 11:8. The mean age of the PUs was 51.6 years (range: 16–81 years). User 19 complained of feeling unwell halfway through the focus group, so FGP5 was completed with only two participants.
https://doi.org/10.1371/journal.pone.0295516.t004
https://doi.org/10.1371/journal.pone.0295516.t005
Of the working PUs, 71% stated they also use the prosthesis in their leisure time, whereas 29% do not. 83% reported using their prosthesis during work. On a scale from 1 to 10 (10 = maximum satisfaction) PUs reported a mean satisfaction of 5.7 (±2.4) for the weight, 5.0 for the noise (±2.4), and 5.0 for the appearance (±2.7) of their prostheses. This data was not collected for the purpose of generalization but to provide an overview of participants’ attitudes towards their prostheses.
After reducing the material and working out its content, three topics were identified that reflect the foci of the participants:
- Requirements for the prosthesis
Requirements on external conditions
Individual factors.
These topics are presented with their subsumed main categories. These main categories were identified from the dataset based on how frequently they were mentioned. In the results, the categories are underlined and accompanied by a citation from just one subject as an example.
Prosthesis requirements
The main categories of this topic are prosthesis design, prosthesis construction, prosthesis appearance, a feedback system, and prosthesis functionality.
Prosthesis design
In all FGs except FGC3, prosthesis weight is considered too high and a lighter weight is preferred.
„And also a question of the socket. If the prosthesis socket is worked accordingly, the weight is not felt that much (OT 1).”
Some subjects (FGP1, FGP2, FGP3, FGC1, FGC2) consider battery life insufficient; others disagree and consider it sufficient. They explain that this apparent contradiction was because battery capacity relates to the power consumption of the prosthesis, prosthesis type and frequency of use, and that these requirements can be very different. Some participants (FGP1, FGP3, FGC1) want to be able to replace the battery themselves.
„The battery capacity, at least in this hand, uh, I think it has a way bigger power consumption than an i-limb hand and therefore is stronger. That’s an advantage and also a disadvantage as the power consumption is way bigger and after half a day the battery is flat (User 13).”
Another important aspect of prosthesis design is the prosthesis socket, especially the socket fitting. The socket should not cause any pressure points and fit tightly despite moisture (FGP2, FGP5, FGC3). It should also be stable (FGP4, FGP2) and easy to independently put on and remove (FGP1, FGP4, FGP5, FGC3).
„The socket is the most important thing, yes (OT 3).”
Prosthesis robustness is discussed in all eight groups. FGC3 and FGP2 emphasize that robustness is more important than a large range of functions.
„Uh the most important thing is a reliable and robust prosthesis (User 4).”
In four groups (FGP1, FGP3, FGC1, FGC2) the wish for a waterproof prosthesis is expressed. Also, the electrodes of electrically controlled prostheses are discussed. FGP1 and FGP2 participants report malfunctions depending on the temperature and humidity of the upper limb stump.
„When I’m working hard and start to sweat, the electrodes start to get wet and I get erratic actions or I have to turn them off (User 1).”
The last prosthesis design aspect relates to the material of the prosthesis glove. Six focus groups (FGP1, FGP2, FGP4, FGP5, FGC1, FGC3) mention that resistance to dirt and contamination of the glove is relevant. Difficulties in cleaning the gloves, the associated additional costs of cleaning and hygiene, and the resulting need for frequent replacements are.
„Actually, I can only add. On the one hand, there is this dirt, which is very unpleasant, that the glove gets dirty, you then have to replace the whole thing, which is very expensive and uh the same applies to hygiene (User1).”
Prosthesis construction
An important aspect here is the distribution of the prosthesis weight. The desire is for the weight of the prosthesis to be shifted proximally, i.e. close to the stump. The therapists remark that muscle-strengthening muscle is important when such a shift is made.
„Yes, I always ask myself why not build a hand and put the motor closer to the stump via a shaft. Yes, where one simply says: reduction of distal weight (OT 1).”
Prosthesis appearance
The relevance of prosthesis appearance is mentioned in seven of the eight focus groups (FGP1, FGP2, FGP3, FGP4, FGP5, FGC1, FGC2). Almost all participants desire a prosthesis with a physiological appearance that goes unnoticed, also under clothing. However, a few PUs prefer a conspicuous prosthesis that is unique and can still look technical.
„What I think is important: It may look technical, but it should have a reasonably chic design (User 13).”
Feedback system
Various aspects of a possible feedback system are discussed. One aspect is the relevance of such a system. Five groups (FGP1, FGP4, FGP5, FGC1, FGC2) state that sensory feedback would be useful. However, some participants also think that a feedback system is unnecessary since optical and auditory control could compensate for much sensory loss.
„Well, apart from hot and cold, I always get feedback somewhere. Because I hear my engine. And I grab (Interviewer: Yes.) And how, I also hear how tightly I grip. You’ll hear that at some point. You have that in your ears (User 7).”
Another aspect is the impact of a feedback system on the personal environment. For example, it is noted that such a system reduces the risk of injury to other people as the grip strength when handling small children can be better assessed.
„Yes, it has already happened. That’s why. So, I grabbed him (the son) by the arm. And I didn’t want to hold it so tight. [omitted]. if they are still a small child, then better without a prosthesis (User 5).”
Implementing such a system is the last aspect. The possibility of an acoustic signal as feedback on the grip strength (FGP1, FGP2, FGP3, FGC1, FGC2) or temperature (FGP2, FGP5, FGC2, FGC3) is suggested. Furthermore, FGP3 repeatedly state that feedback on proprioception could be useful. A feedback signal in the form of vibrations is also discussed (FGP3, FGC1).
Functionality of the prosthesis
Grip strength is a highly discussed aspect of functionality. In four groups (FGP3, FGP4, FGP5, FGC1) greater grip strength is mentioned as important. FGP3 and FGC3 state that grip strength is insufficient. Grip velocity is brought up here as some users (FGP2, FGP3) consider it too slow. Even velocity regulation through Dynamic Mode Control (DMC) is insufficient. The wish to be able to quickly switch between different grips is also mentioned (FGP5, FGC2, FGC3).
„Too little power, it’s just-. Well, a Touch Bionic hand just doesn’t have enough strength to hold something, no matter what, in such a way that they … are especially when it’s fine grips, the strength uh the fine grips that require strength. A Touch Bionics hand can’t do that because the thumb turns to the side, so it was like that with mine (User 13).”
In all groups except FGC1, participants state the relevance of a large range of functions. Desired functions are distinguished into gripping, holding, and fine-motor functions, which are all considered important. Gross motor function is mentioned less often. In the FGP2 group, the wish for simultaneously performed grips is expressed. Also, users of passive prostheses desire different grip possibilities. Requests for automatized grip patterns (FGP2), the possibility of touchpad handling (FGP1, FGP5) as well as the controlling of different grip patterns via app (FGP5, FGC3) come up. Apart from the grip capabilities, participants mention malfunctions of the prosthesis and activities that cannot be performed with the prosthesis. Examples stated here are electromagnetic interference (FGP1, FGP2) or uncontrolled movements of the prosthesis (FGP1, FGP2, FGP3, FGC1). According to the technicians, a poorly adjusted socket can be the cause of such incorrect controls.
„And, as the gentlemen here have already said, the prosthesis does what it’s not supposed to. (Everyone laughs) It’s like sometimes a finger goes up and down, even though he really can’t do anything about it. Sounds weird, but still, yes, I think they have a life of their own (User 10).”
Various application areas are identified as desirable for the prostheses. Operating vehicles (FGP1, FGP2, FGP3, FGP4, FGP5, FGC1, FGC2) and housekeeping (FGP1, FGP3, FGC1, FGC2) are frequently mentioned, and the use of cutlery or tools is also viewed as desirable.
Another aspect of functionality are the technical requirements for the prosthesis. In FGP1, FGP3, and FGC1, reliability of the prosthesis is considered more important than a modern appearance or large range of function. Further aspects are the wishes for intuitive movements (FGP1, FGP4, FGP5, FGC3), mechanical stability (FGP3), and the demand for a simple control of the prosthesis (FGP2, FGP5, FGC3). Automatic regripping of the prosthesis at physical contact is viewed negatively (FGP2, FGC3). Some participants also articulate the desire to individually adapt the prosthesis’ range of functions. In four groups (FGP1, FGP3, FGP4, FGP5), the participants desire more flexible fingers, whereas in another four groups (FGP1, FGP4, FGP5, FGC2) pro- and supination capabilities were requested.
The main categories here include prosthesis fitting and service.
Prosthesis fitting
In almost all groups, costs associated with owning a prosthesis are criticized.
„And what I would wish for is that the uh (short pause), how should I put it, that cheaper solutions would be found (User 13).”
Another fitting aspect is related to therapists, technicians, and physicians. While users underline the importance of communication with care staff (FGP3), technicians and therapists underline the relevance of interdisciplinary collaboration between professional groups. Technicians also highlight the importance of therapeutic care of the patients but are also critical about the lack of experienced therapists active in prosthetics (FGC2).
„But here we have the actually largest deficit in this collaboration. (Interviewer: Where?) So good prosthetists that can deal with the technology there are enough of. Physiotherapists that can simply and comprehensively get to work without one of us supervising, uh yeah… I would say there is hardly anyone (OT 1).”
A last aspect here is the equipment of the persons concerned. Five groups (FGP3, FGP4, FGC1, FGC2, FGC3) frequently mention that the type of prosthesis needs to be individually customized to users. Furthermore, users request substitutional supply (FGP3, FGC1, FGC3), especially when the prosthesis is defect. It is suggested that prostheses no longer used should be returned to care providers so that these could be offered as a replacement or testing supply for other users.
Excessive waiting times during prosthesis repair are mentioned.
„And the service times should be well under six weeks (User 7).”
The lack of available information about technical innovations is criticized. There is also criticism of the unrealistic advertising available on the internet, which arises false expectations among users. On top of that, trial prosthesis supply should be available for more than two weeks to ensure that a suitable prosthesis is identified and to maximize prosthesis use. Furthermore, local service centers should be able to perform standard maintenance (FGP1, FGP3, FGC2, FGC3).
The main individual factors categories are psychosocial factors, somatic factors, and the prosthesis use.
Psychosocial factors
In this category, a frequent subject of discussion is the external effect of the prosthesis. Impressions differ between greater acceptance for the arm stump or for the prosthesis. Opinions vary particularly in the case of children. Regarding sports, it is also stated that peers are afraid of being injured by the prosthesis. Five focus groups (FGP1, FGP2, FGP3, FGP5, FGC3) state that the prosthesis completes the body image. Participants also state that they seem disabled without the prosthesis (FGP3, FGP4, FGP2).
„The positive thing is that others don’t immediately notice that you are disabled (User 14).”
In addition, participants report that everyday life is not possible without the prosthesis as it enables independence and self-determination.
„Because without a prosthesis (short pause) our quality of life is zero (User 11).”
Somatic factors
In this category, the focus is on the influence of the stump. Five groups (FGP1, FGP2, FGP3, FGC1, FGC3) mention, that amputation level influences prosthesis functionality. The disadvantages associated with a short stump are emphasized, i.e. a short stump aggravates distal gripping (FGP4, FGC2, FGC3). Participants of FGP1 also state that electrode contact depends on stump shape.
Use of prostheses
Prosthesis use is another frequently addressed issue. Participants in the PP group repeatedly criticize the prosthesis as uncomfortable, superfluous, and disruptive and that it must offer benefits in order to be used. Within this group, it is repeatedly mentioned that there is no interest in technical innovations on the prosthesis market.
„It’s just plain and simply superfluous. As long as you have one hand that you can really do something with, you don’t do anything with it (note: with the prosthesis). Then it’s just plain and simply a burden, the thing just gets in the way (User 14).”
Four groups (FGP4, FGP5, FGC2, FGC3) state that dysmelia patients are used to coping without a prosthesis and that they see it more as a tool than as a replacement hand. Many participants underline that effort and benefit must be balanced when using the prosthesis (FGP4, FGP5, FGC2) and that awareness of the advantages of the prosthesis influences the acceptance (FGC1, FGC3).
„Yes, and that (note: the myoelectric prosthesis) totally fascinated me, which is why I had it demonstrated to me because I was totally fascinated. But, as I said, even then the point hadn’t been reached, uh that uh well, from my perspective, you really have added value. The disadvantages: costs, weight, lack of robustness, sensitivity, etc. were just higher than the benefit (User 16).”
Some participants express the importance of being independent from the prosthesis (FGP1, FGP4, FGP5). For scope of use, it is often stated that the prosthesis is only removed while in the water or sleeping (FGP1, FGP3, FGP5, FGP2) and that use of prostheses in leisure time is also highly relevant (FGP4, FGC2). However, other participants state (FGP4, FGP5) that they only use their prosthesis at work.
The sociodemographic data of prosthesis users is in accordance with the overall data of upper extremity amputees, as most of them are male and trauma is the most common reason for amputation [ 5 , 16 ]. The results are at first discussed in relation to the research questions followed by an overview of mentioned aspects that have been addressed in the SoftPro project.
1. What are the most important requirements for users and professionals?
The main requirements mentioned are prosthesis weight, battery capacity, fitting and manufacture of the socket, reliability, robustness, glove’s susceptibility to dirt, prosthesis appearance, grip strength and velocity, and possibilities for fine and gross motor functions as well as physiological movements. These aspects were frequently mentioned, indicating their high relevance for the participants. Some external factors were also frequently mentioned, such as demands for an affordable prosthesis, interdisciplinary care, the possibility of trial fittings, and a fast service close to home.
2. Have technical innovations in recent years changed the requirements of users and professionals?
Many points of criticism still coincide with criticisms identified in comparable studies in the past. Notable criticisms are prosthesis weight, susceptibility of the prosthesis glove to dirt, fitting of the socket, and prosthesis robustness and appearance [ 5 , 17 – 25 ]. Also, criticism of insufficient grip strength and grip speed persist [ 5 , 8 , 9 , 19 , 22 , 26 ]. That most problems mentioned by patients with prosthetic hands and arms appear unchanged after two decades demonstrate the prosthetic industry’s inability to adequately address these issues. Therefore, manufacturers need to incorporate development and innovation processes that take users’ needs and professionals’ recommendations into account. As sometimes happens in other technical fields, new developments in hand prosthetics appear to have a partially detrimental effect on initially advantageous developments. One example is the improvement of battery capacity, which is canceled by the increased power consumption of multi-articulating prostheses to meet the requirement for movable fingers [ 4 , 17 ].
3. Which unfulfilled requirements of past prosthetic devices can be addressed with future prosthetic devices?
The participants formulated specific requirements for prostheses that can be directly addressed through new technical developments. These include requirements for prosthesis design (weight, battery, socket, electrodes, robustness, prosthetic glove), structure, and appearance, a possible feedback system, and prosthesis functionality (grip strength, grip speed, grip options, intuitive grip patterns). Although these requirements can be addressed directly with new developments in prosthetics, an improved prosthesis does not guarantee successful use. For example, some users of passive prostheses find the prosthesis annoying and superfluous and are therefore not interested in new types of prostheses. In such cases, education should be carried out first, since—as participants in FGC1 and FGC3 mentioned—awareness of the advantages of the prosthesis influences acceptance.
The emphasis on relevance of the interaction between a well-functioning prosthesis and a well-developed infrastructure for successful prosthesis use has only been mentioned occasionally in other studies. This could be because previous research either focused on the prosthesis [ 7 , 8 ] or on the psychosocial aspects [ 2 ] but not on the infrastructure, which also plays an important role for users.
In general, additional solutions for care and service should be developed, which besides education, include financing, alternating and test supplies, and reduced maintenance times. In addition to prosthesis and infrastructure requirements, this study shows how strong the influence of users’ individual psychological and physical prerequisites is on prosthesis use, which is why new developments should consider these. Further research is also necessary and should address whether an improved production of currently available prostheses (e.g., better adaptation of the socket, reorganization of individual components, etc.) would be enough to improve their functionality. A quantitative investigation based on this study to assess the statistical significance of the identified requirements would also be useful. Finally, it should be investigated whether close interdisciplinary support could improve the influence of psychosocial factors on prosthesis use.
In the aforementioned SoftPro project, an industrial design method with multiple strands was employed as the foundation for transferring a robotic end effector gripper into a prosthetic hand, based on the structure and kinematics of the human hand. This design method was based on a user centred approach and for this reason encompassed various aspects, including the consideration of practical issues and the desires of amputees, users, and experts in the field of upper limb prosthetics from the present focus group study.
The authors were somewhat taken aback by the long-standing inadequacies, which they encountered during the development process and were already known for years. Therefore, the development of the prosthetic hand focused on the aspects most frequently mentioned here. As a result, the primary focus during the development of the SoftHand Pro (Version 1) was on achieving a combination of fundamental and advanced grasping functionality, precise and reliable EMG control, adaptable grasping capabilities (ranging from firm to gentle and also speed-adjusted grasping), the ability to manipulate objects of varying sizes (without requiring switching processes), complete mobility of fingers and thumb (eliminating rigid finger segments through the use of soft robotics), weight reduction of the prosthetic hand (m = 520g through lightweight construction) and the prosthetic arm (optimized battery positioning), and compatibility with other myoelectric systems. Additionally, considerable attention was given to ensuring comparatively low manufacturing costs.
As part of the further development within the Technology Readiness Level framework, the SoftHand Pro (Version 3) was designed. This version aimed to improve upon the second hand version, focusing on enhancing the cosmetic appearance through anatomically optimized hand and finger proportions, improving the aesthetic appeal through an optimized cosmetic glove that facilitates easier and thorough cleaning of the hand and enables touch screen operability, improving the grasping forces, introducing passive wrist mobility, employing more robust and less error-prone mechanical and electrical components, enabling the ability to eat with cutlery, further reducing the overall weight (m = 290g), and achieving nearly complete water resistance.
Ultimately, a fundamental aspect was also ensuring a lifespan of system usage compatible with the patients’ needs. In this regard, the internal implementation scheme and the design of the finger’s elastic components were completely revolutionized. This has extended the system’s functional life from a few thousand cycles to hundreds of thousands of opening and closing cycles.
Limitations
Although the number of participants in this study is comparable to other qualitative studies in this area, the results cannot be generalized. Rather this study should be viewed as an extensive collection of relevant aspects for developing prostheses. The group sizes varied between three and six participants. Although FGP5 started with three participants, only two were left at the end. In the focus groups of the technicians and therapists, some of participants already knew each other beforehand, which might have influenced their statements since a certain distribution of roles already existed. The moderator therefore ensured that all participants had the opportunity to speak. Finally, the number of group participants per focus group varied, which might have influenced the frequency of mentions.
In this study, focus groups on the improvement of prosthetic design and care were conducted with Pua, Ots, PTHs and OTHs and qualitatively evaluated using summarizing content analysis. Focusing on the development of future prostheses, three main topics relevant for improving function and reducing rejection were identified: a properly functioning prosthesis, the infrastructure and users’ psychological and physical prerequisites. The most commonly mentioned technical deficiencies with regard to currently available hand prostheses from the users’ perspective were the robustness of the prostheses, lacking possibilities for fine and gross motor functions as well as physiological movements, and the glove’s susceptibility to dirt. This information is already used in the development of the SoftHand Pro and could play a key role for manufacturing stakeholders in significantly improving the quality level for amputees through rapid and effective technical enhancements. A successful technical addressing of these deficiencies should be in the best interest of such companies, as it presents the opportunity to generate long-term customer loyalty.
The interaction between a well-functioning prosthesis and a well-developed infrastructure was identified as an important influence on successful use. Additional solutions for supply and service are therefore needed. Most problems identified in the literature in recent decades are still not addressed today. Therefore, the prosthetic industry should try to pay more attention to users’ needs and professionals’ recommendations. Moreover, individual psychological and physical prerequisites for prosthesis use should be considered in new developments.
Supporting information
S1 appendix. anonymized transcripts in german language..
https://doi.org/10.1371/journal.pone.0295516.s001
Acknowledgments
The authors would like to thank all volunteers for their efforts and patience during the study, Jens Froböse and Ingo Schröter from John + Bamberg GmbH & Co. KG for helping with prosthetic user recruiting, Alina Obermeier and Benjamin Fleischer-Lück for assistance in data collection and processing, and Crystal Emonde for spell and grammar check this paper.
- View Article
- Google Scholar
- PubMed/NCBI
- 15. Mayring P, Fenzl T. Qualitative Inhaltsanalyse. In: Baur N, Blasius J, editors. Handbuch Methoden der empirischen Sozialforschung. 2., vollständig überarbeitete und erweiterte Auflage. Wiesbaden: Springer VS; 2019. p. 633–48 (Springer eBook Collection).

An official website of the United States government
The .gov means it’s official. Federal government websites often end in .gov or .mil. Before sharing sensitive information, make sure you’re on a federal government site.
The site is secure. The https:// ensures that you are connecting to the official website and that any information you provide is encrypted and transmitted securely.
- Publications
- Account settings
Preview improvements coming to the PMC website in October 2024. Learn More or Try it out now .
- Advanced Search
- Journal List
- Scientific Reports

Clinical evaluation of the revolutionizing prosthetics modular prosthetic limb system for upper extremity amputees
Kristin e. yu.
1 Center for Rehabilitation Sciences Research, Henry M. Jackson Foundation for the Advancement of Military Medicine, Bethesda, MD USA
Briana N. Perry
Courtney w. moran.
2 Applied Physics Laboratory, Johns Hopkins University, Laurel, MD USA
Robert S. Armiger
Matthew s. johannes.
3 Outrider.ai, Golden, CO USA
Abigail Hawkins
4 Uniformed Services University of the Health Sciences, Bethesda, MD USA
Lauren Stentz
Jamie vandersea.
5 Medical Center Orthotics & Prosthetics, Silver Spring, MD USA
Jack W. Tsao
6 University of Tennessee Health Science Center, Memphis, TN USA
7 Children’s Foundation Research Institute, Le Bonheur Children’s Hospital, Memphis, TN USA
Paul F. Pasquina
Associated data.
Individuals with upper extremity (UE) amputation abandon prostheses due to challenges with significant device weight—particularly among myoelectric prostheses—and limited device dexterity, durability, and reliability among both myoelectric and body-powered prostheses. The Modular Prosthetic Limb (MPL) system couples an advanced UE prosthesis with a pattern recognition paradigm for intuitive, non-invasive prosthetic control. Pattern recognition accuracy and functional assessment—Box & Blocks (BB), Jebsen-Taylor Hand Function Test (JHFT), and Assessment of Capacity for Myoelectric Control (ACMC)—scores comprised the main outcomes. 10 participants were included in analyses, including seven individuals with traumatic amputation, two individuals with congenital limb absence, and one with amputation secondary to malignancy. The average (SD) time since limb loss, excluding congenital participants, was 85.9 (59.5) months. Participants controlled an average of eight motion classes compared to three with their conventional prostheses. All participants made continuous improvements in motion classifier accuracy, pathway completion efficiency, and MPL manipulation. BB and JHFT improvements were not statistically significant. ACMC performance improved for all participants, with mean (SD) scores of 162.6 (105.3), 213.4 (196.2), and 383.2 (154.3), p = 0.02 between the baseline, midpoint, and exit assessments, respectively. Feedback included lengthening the training period to further improve motion classifier accuracy and MPL control. The MPL has potential to restore functionality to individuals with acquired or congenital UE loss.
Introduction
The incidence of limb loss has increased in the last decade due to combat injuries in the military population and non-traumatic causes of amputation in the civilian and aging veteran populations such as peripheral vascular disease, diabetes, and malignancy. As of April 2019, 297 service members had sustained upper extremity (UE) limb loss in Operation Iraqi Freedom (OIF) and Operation Enduring Freedom (OEF) alone, representing 17.3% of amputees treated at Military Treatment Facilities (MTFs) 1 . Individuals with UE limb loss experience difficulty performing activities of daily living (ADLs) such as performing acts of personal hygiene (i.e. combing hair, brushing teeth, etc.), dressing, and preparing and eating meals. Prosthetic use following limb loss can restore function and increase quality of life. An ideal UE prosthesis would confer the ability to smoothly manipulate objects in the environment with fidelity, appropriate grip strength, dexterity, anthropomorphism, and fine motor control in a coordinated manner. In this way, the prosthesis would effectively replicate characteristics of the human hand with respect to shape, function, perception, and incorporation into the user’s sense of self 2 . Patients with UE limb loss cited device weight, durability, reliability, dexterity, and limited sensory feedback as factors for UE prosthesis rejection and abandonment 3 – 5 .
Upper limb prostheses can be divided into passive and active devices. Conventional body-powered prostheses offer simplicity, durability, lightness of weight, and tension feedback to the user with control of basic hand grasps 3 . Active devices—with myoelectric prosthetic hands and neural processing systems—mark a significant technological advancement over conventional body-powered prostheses. Several poly-articulated myoelectric prosthetic hands are currently on the market, including the Michelangelo ® , i-Limb ® , and Bebionic ® Hands, which enable multiple degrees of freedom (DOF) of control for three to five unique grasps 6 . Commercial hand prostheses are characterized by diminished grip strength and limited finger dexterity and often require manual correction of hand and finger position to switch between grasps, despite being poly-articulated 7 .
In addition to the benefits of poly-articulated upper extremity devices in actuating a greater number of grasps than achievable with body-powered prostheses, progress has been made with respect to prosthetic control schemes. The majority of myoelectric devices rely upon direct control schemes that function according to signal amplitude-based paradigms 8 , 9 . Myoelectric control classically operates via an on/off paradigm with long training requirements prone to degradation and rarely allows for simultaneous control over multiple DOF. Motion switching is enacted through the control of opposing muscle groups and becomes more difficult with increases in component and motion sequence complexity 10 . Furthermore, these control schemes lack corresponding interfaces through which intuitive motor directives can be connected with movement of the prosthesis in real-time 6 . By contrast, pattern recognition enables users to control multiple DOF simultaneously and independently for more seamless and facile control of multiple movement classes 10 . However, signal degradation may still result due to changes in arm and electrode position, fatigue, and signal cross-talk, with lengthy training requirements 6 . Simon et al. conducted a home trial of trans-radial amputees who controlled multiple-DOF prostheses via a direct control or pattern recognition paradigm and found no significant difference in the number of grasps manipulated between control schemes. However, direct control users spent the majority of their time in a single grasp compared to pattern recognition users, who divided their time between three different grips 9 . The authors theorized that pattern recognition control affords more intuitive selection of and switching between trained motion classes 9 . Hargrove et al. reported similar findings from a randomized controlled trial of trans-humeral amputees with Targeted Muscle Reinnervation (TMR) who performed tasks that required three DOF—the Box and Blocks test, a clothespin relocation task, and a block stacking task—under direct or pattern recognition control schemes 11 . The authors found that real-time pattern recognition control outperformed and was more quickly configured than direct control in these dexterous tasks 11 . Qualitative reports from participants revealed a significant preference for pattern recognition over direct control due to user-perceived ease of use and greater consistency with respect to prosthetic control.
The Modular Prosthetic Limb (MPL) was developed by the Johns Hopkins University Applied Physics Lab (JHU APL) as part of the Defense Advanced Research Projects Agency (DARPA) Revolutionizing Prosthetics initiative. The MPL’s pattern recognition system enables user control over an increased number of actuating joints. The MPL system has 26 independently controllable movement classes (DOF) controlled by 17 independent motors. The system classifies these signals in real-time and allows customization of user movement classes for individual digit, arm and wrist joint control, and coordinated grasping and arm motion. Through this control scheme, a user visualizes and practices moving his or her missing limb, during which the system recognizes and matches the elicited patterns of muscle activation with the selected motion classifier, thus yielding intuitive, personalized, and repeatable prosthetic control 12 – 14 . The MPL’s control interface utilizes pattern recognition for individual joints and integrated reduced order control (ROC). ROC allows for the combination and integration of complex multi-joint motions to simplify user control over motion classes. An example of ROC with respect to the MPL’s individual finger dexterity allows users to achieve a cylindrical-shaped grasp by visualizing the entirety of the cylindrical grasping motion during training. By training the integrated motion class, users can more easily achieve the target grasp without commanding each finger to close independently to the desired extent (e.g. around a glass) each time. Other unique, innovative features of the MPL include: 360°/s joint speed, multi-articulating fingers, a four DOF thumb, finger spreading via abduction and adduction, joint sensors for position, velocity, and torque, integrated limb control system hardware and software, and a three DOF modular wrist (i.e. rotation, flexion/extension, and abduction/adduction). In addition, the MPL can achieve an anthropomorphic curl strength of 45 ft.-lbs. and maximum elbow joint speeds of 120°/s and includes a three DOF shoulder (i.e. flexion/extension, abduction/adduction, and internal/external rotation) and one DOF elbow 15 . Through these features, the MPL seeks to restore the functionality and kinematics of the human arm and hand to individuals with varied levels of upper extremity limb loss.
Although the MPL can support a modular and diverse set of input control sources, we examined only non-invasive signal acquisition from surface electromyograms (sEMG) as the driver for advanced pattern recognition control. Advances in myoelectric signal processing and TMR have made it possible for individuals with limb loss to identify and discriminate between intended neuromuscular control patterns. TMR is a surgical procedure in which nerves from the residual limb are transferred to other anatomic locations to reinnervate new muscle targets 16 . These residual nerve signals are amplified, allowing for facilitated and more intuitive control of prostheses 16 . The MPL was configured for study use with a socket interface and osseointegration (OI) 17 . OI is the direct joining of living bone and a load-carrying implant, with dynamic interactions at the tissue-implant interface 18 . OI entails the surgical introduction of the implant to bone and bone remodeling through which rigid fixation is achieved and maintained during functional loading 18 .
This study evaluated the performance, feasibility, and acceptance of the MPL in clinical scenarios simulating ADLs. It further sought to assess the number of optimally controlled DOF that can be achieved with this advanced prosthetic control system. There were two study phases: a virtual training phase (Phase I), followed by socket fabrication and electrode integration, and a clinical training phase (Phase II). Through the virtual training sessions, participants were introduced to non-invasive pattern recognition control, which they later utilized to control the physical MPL prosthesis over 12 clinical training sessions. Participants completed formal qualitative and functional assessments during the baseline, midpoint, and final clinical training sessions.
This primary study objective was to evaluate the performance and feasibility of the MPL in clinical scenarios simulating activities of daily living (ADLs). The secondary objective was to determine the number of optimally controlled MPL DOF at different levels of UE loss. We hypothesized that: (1) a greater number of MPL motion classes would be controlled by participants over time, with improvement between the first and last clinical sessions; (2) participants would improve in their ability to perform functional assessments with the MPL between the first and last clinical sessions; and (3) participants will report greater self-perceived ease in performing simulated ADLs with the MPL over their conventional “home” prostheses by the end of the training period due to the MPL’s intuitive control scheme and advanced features.
All procedures were performed at Walter Reed National Military Medical Center (WRNMMC) following approval by the WRNMMC Institutional Review Board. All research procedures were performed in accordance with Human Research Protection Program guidelines through the WRNMMC Department of Research Programs (DRP). The study is registered at ClinicalTrials.gov ( {"type":"clinical-trial","attrs":{"text":"NCT02887690","term_id":"NCT02887690"}} NCT02887690 , 02/09/2016). The study spanned from February 21, 2014 to September 7, 2017. Study length ranged from six to seven weeks depending on the time needed for socket fabrication and integration.
Participants
This study recruited participants between 18- and 65-years-old with unilateral or bilateral UE loss and was approved to enroll up to 24 individuals in Phase I and 12 individuals in Phase II (Supplementary Materials 1 ). Recruitment occurred via referral from clinicians at WRNMMC and Medical Center Orthotics & Prosthetics (MCOP).
Participants were included if they could follow study instructions, had no prior history of vertebral disk disease, sciatica, or radiculopathy, and were medically cleared to use a prosthetic device. Participants were excluded if they had traumatic brain injury, a significant Axis I/II diagnosis, a pacemaker, uncontrolled systemic disease, or any other medical condition that might put the subject at risk. Participants were informed of the potential risks and benefits of study participation and their rights as study participants, and given time to ask questions of the study team prior to providing informed consent. Written informed consent was obtained from all participants in accordance with WRNMMC guidelines. Participants were screened using the Test of Memory Malingering in order to ensure adequate participant effort and ability to follow directions; scores lower than 42/50 were exclusionary.
Participants were compensated for their time and travel with a $50 giftcard unless they were an active duty service member or federal employee.
Phase I: virtual training
Phase I consisted of four, one-hour virtual training sessions. Participants trained with the Virtual Integration Environment (VIE), a virtual reality simulator for UE prosthetic training 19 . An open-source version of the code was created as part of The Open Prosthetics Project ( http://openprosthetics.org/ ) 20 in affiliation with the Johns Hopkins University Applied Physics Laboratory (JHU APL) and is available at https://bitbucket.org/rarmiger/minivie . The VIE allows individuals to direct the movements of a virtual avatar using surface electromyogram (sEMG) signals captured from their residual limbs, digitized through an electrically-isolated data acquisition system, and filtered using signal analysis algorithms. Our laboratory has previously described the utility of the VIE in training individuals with upper extremity amputation in this pattern recognition platform for prosthetic control 21 , 22 . Motions included hand open, wrist flexion, extension, pronation, and supination, several user-selected grasps, “no movement”, and elbow flexion and extension with trans-humeral amputation. Depending on amputation level, electrodes were contained in a non-adhesive Myoband (Thalmic labs, Waterloo, Ontario) or using custom wired bioinstrumentation amplifiers as part of the MPL system. Signal feature extraction and motion classification occurred using machine learning-based pattern recognition software and a Linear Discriminant Analysis classifier. The same software interfaces are used to control the MPL prosthesis 17 , 21 , 22 .
At the end of each session, participants completed a 1-DOF Target Achievement Control (TAC) 23 test that characterized the accuracy with which motion classes were achieved. TAC scores were evaluated with respect to movement completion and path efficiency, which were normalized for the number of grasps trained. Motion completion scores reflect the ability to achieve and maintain a unique pattern of muscle contraction for each motion class over 20 s without signal overlap with other motion class patterns. Path efficiency scores reflect the ability to move a virtual avatar from an initial to target position on the screen. Overshooting and correction for the aberrant motion generates an increased linear motion distance that reduces path efficiency. An average TAC score ≥ 75% was needed to qualify for Phase II.
Phase II: socket fabrication and clinical training
Participants who qualified for Phase II were cast and fit for a socket that was integrated with sEMG electrodes (Liberating Technology, Inc. Holliston, MA, USA). Electrode number and orientation varied according to amputation level and residual limb anatomy. Participants with OI had previously undergone implantation of the Osseointegrated Prostheses for the Rehabilitation of Amputees implant (Integrum AB, Sweden) and trans-dermal Compress™ system (Zimmer Biomet, Warsaw, Indiana) and received a custom connecting piece to affix the MPL to their implant. These participants used the Myo armband for MPL control.
Participants completed 12 MPL training sessions. Each session included motion class training, ADL simulation, and a TAC assessment. The first (i.e. baseline), sixth (i.e. midpoint), and 12th (i.e. exit) sessions included functional and subjective testing suites. At the baseline assessment, participants completed these suites with their home, conventional prostheses. At the midpoint and exit assessments, these assessments were completed using the MPL. Baseline assessments could not be completed for two participants who lacked home prostheses.
The functional tests administered at the baseline, midpoint, and exit assessments included the Box and Blocks (BB) 24 test, Jebsen-Taylor Hand Function Test (JHFT) 25 , and Assessment of Capacity for Myoelectric Control (ACMC) 26 . The BB test pertains to efficiency in repeating a motion and grasp sequence to transfer blocks over a raised barrier. The JHFT evaluates the ability to perform seven ADLs. The number of objects moved per second was calculated to track improvements in device control. The ACMC assesses the effectiveness of myoelectric device use during ADLs—dressing, food preparation, ironing, packing a suitcase, or driving—and was developed and validated for UE prosthetic outcomes testing 27 . The suitcase packing ACMC task was used for all participants.
Subjective metrics included the Upper Extremity Functional Scale (UEFS), Trinity Amputation and Prosthesis Experience Scales-Revised (TAPES-R), and modified Visual Analogue Scale/Short-Form McGill Pain Questionnaire (VAS/SF-MPQ) (Supplementary Materials 2 ) 25 , 26 , 28 . The UEFS measures self-reported functional ability to perform 23 ADLs 25 . The TAPES-R captures the type and length of time since amputation, prosthesis usage, and phantom limb pain. The VAS is a simple, internally consistent, and reliable measure of pain validated for clinical and research use 29 . The SF-MPQ is used to assess pain quality and severity 30 , 31 .
The MPL User Feedback survey (Supplementary Materials 3 ) was written by our research team for the purposes of this study and assessed participant experiences and perceived levels of activity. Questions were selected based on the recommendations of the UE prosthetic outcome measures working group and prior studies that evaluated user prosthetic experiences, functionality, and self-reported levels of ability with prostheses 17 , 27 , 32 , 33 .
Statistical analysis was performed using repeated measures (RM) one-way ANOVAs and two-tailed t-tests in Prism 8.0 (GraphPad Software Inc., LaJolla, CA). ANOVAs were conducted using Bonferroni adjusted alpha levels of 0.0167 to evaluate the three a priori hypotheses. Remaining analyses were performed with statistical significance defined as p < 0.05.
This effort was supported through Uniformed Services University of the Health Sciences (USUHS) Grant HU0001-11-1-005.
21 participants were consented. Eight individuals withdrew prior to Phase I due to scheduling concerns. One participant did not meet study criteria to advance to Phase II. One participant withdrew from Phase II due to scheduling conflicts. 11 participants completed all study procedures (Supplementary Materials 1 ). One participant was omitted due to the use of a translator approved for medical, but not research, services.
Of the 10 individuals included in analyses, 50% had trans-humeral and 50% had trans-radial amputations, including one wrist disarticulation (Table (Table1). 1 ). Three individuals with trans-humeral amputations had OI and TMR. Two individuals with trans-radial amputations had congenital limb absence. One individual with congenital limb loss had used a prosthesis daily for ADLs since birth, as reported through the TAPES-R survey, while the other had never used a prosthesis prior to study enrollment.
Participant demographic and clinical characteristics.
Study participant demographic and clinical characteristics. Of 21 consented research participants, 11 completed all study sessions and 10 were included in final data analysis. Participants with congenital limb absence were excluded from analyses of average time since limb loss and of prosthetic use.
The average time since limb loss and duration of prior prosthetic use, excluding participants with congenital limb loss, were 85.9 months ( s = 59.5) (Table (Table1) 1 ) and 8.6 months ( s = 8.8), respectively.
12 participants met the TAC cutoff to advance to Phase II, with average scores of 75–100% across motion classes in the Virtual Integrated Environment. After populating the pattern recognition software with sEMG signals corresponding to distinct virtual motion classes, as prompted by on-screen commands, participants performed this one DOF TAC to classify motion accuracy. These participants achieved the requisite scores for study advancement within the allotted four session Phase I study period. Virtual training data was used solely to identify participants with sufficient pattern recognition paradigm mastery to control the physical MPL prosthesis during the clinical training period and were not analyzed as part of this study.
Individuals who regularly used a home prosthesis (n = 9) controlled an average of three motion classes at baseline. Home prostheses ranged from conventional devices such as the body-powered hook to myoelectric hands. The initial motion classes trained with the MPL were hand open and “no movement”. On average, participants were able to control six and eight MPL motion classes by the midpoint and exit assessments, respectively. The maximum number of motions controlled with the MPL was 12, including five distinct grasps. There were no significant differences between the number of motions controlled by participants with and without OI/TMR and between amputation levels (Fig. 1 ).

Average number of motions controlled by amputation level, defined as transhumeral, transradial and wrist disarticulation, with participants’ conventional prosthesis (current) compared to the MPL.
At the beginning of Phase II, participants were asked about upper extremity motions and activities significant to them that they had not been able to perform satisfactorily with their previous or current prostheses. After participants demonstrated successful manipulation of common objects such as pegs, foam shapes, and stackable cones with the MPL during clinical sessions, they practiced performing ADLs, which were customized according to their expressed task preferences. These ADLs included drinking from mugs, eating with utensils, reaching for overhead objects and stirring pots during meal preparation, writing in cursive, lacing, tying, buttoning a shirt, and playing catch.
TAC scores for individual and combined motion sets improved for all participants across time. A significant improvement in normalized completion scores was observed by RM one-way ANOVA over time, with mean (SD) scores of 3.7 (1.2), 4.1 (1.7), and 6.3 (1.7) at the baseline, midpoint, and exit assessments, respectively, p = 0.003. Improvement in normalized path efficiency was also observed by RM one-way ANOVA over time, with mean (SD) scores of 2.5 (0.9), 2.9 (1.1), and 4.2 (1.5), p = 0.004, respectively. Simultaneous control of multiple motions slowed task completion by compelling participants to reliably execute distinct patterns of muscle activation and switch between them in an appropriate sequence. Since continuous improvements were observed, the maximum number of controllable motions could not be determined. Participants demonstrated the greatest levels of prosthetic control over the tripod, spherical, tip, and cylindrical grasps.
No significant differences were found between the number of BB blocks transferred with the MPL at the exit assessment relative to the number transferred using home prostheses during the baseline session (Fig. 2 ). BB improvements with the MPL between the midpoint and exit assessments were not significant.

Number of boxes moved during the Box and Blocks assessment for each participant during the baseline, midpoint, and exit sessions. The Box & Blocks task was performed in triplicate during each session and the number of blocks moved was averaged. Two participants (MPL_14 and MPL_20) did not have or regularly use a conventional prosthesis at the time of study participation and did not perform the Box & Blocks task as part of a baseline assessment.
9 of 10 participants improved in JHFT tasks over time. Individuals with trans-radial amputations outperformed those with trans-humeral amputations with both conventional prostheses and the MPL (Fig. 3 a,b). Improvements in JHFT scores over time were not statistically significant between and within individuals ( p > 0.05). The JHFT tasks with the highest scores were Small Common Objects and Page Turning, while those with the lowest scores were Simulated Feeding and Heavy Objects, regardless of prosthesis used. Of note, the Simulated Feeding task required participants to maintain a steady grip of their choosing on a metal spoon, which was used to scoop individual beans into a can. Participants expressed subjective difficulty in positioning and balancing beans while maintaining their grip on the spoon, which requires sustaining a closed hand grasp motion class throughout the planned motor sequence.

( A ) The time to complete each task of the Jebsen-Taylor Hand Function Test for participants with trans-radial amputations using the MPL. Participants completed these tasks using grasps and motion classes he or she had trained in previous clinical sessions with the MPL. ( B ) The time to complete each task of the Jebsen-Taylor Hand Function Test for participants with trans-humeral amputations using the MPL. Participants completed these tasks using grasps and motion classes he or she had trained in previous clinical sessions with the MPL.
ACMC performance improved for all participants over time by RM one-way ANOVA, with mean (SD) scores of 162.6 (105.3), 213.4 (196.2), and 383.2 (154.3), p = 0.02 between the baseline, midpoint, and exit assessments, respectively (Fig. 4 ). No significant ACMC variation was discerned with respect to age, duration and level of amputation, TAC motion completion or path efficiency scores, or duration of prosthesis use.

Assessment of Capacity for Myoelectric Control (ACMC) scores for each participant by percent ability, normalized to the number of DOF controlled during the session.
UEFS and TAPES-R scores did not vary significantly between the initial and final sessions ( p > 0.05), including participants with congenital limb absence. Overall, individuals with trans-humeral amputations reported less activity restriction than individuals with trans-radial amputation (mean difference (95% CI) 0.2 (0.007 to 0.4), p = 0.01).
Four individuals reported that the MPL was more functional than their current prostheses—which ranged from a body-powered hook to the iLimb ® and BeBionic ® hands—and cited the ease of changing grasps, ability to pick up larger objects, and durability of the prosthesis as benefits. Three participants felt that at their current level of training (i.e. 12 sessions with the physical prosthesis), the MPL was more useful than their existing prostheses, while three felt that they needed additional training time with the MPL for it to become more useful than their home device. Six participants felt that they needed more practice to reach optimal performance with the MPL in performing routine ADLs and seven participants felt that they could be comfortable using the MPL on a daily basis within two months of study completion. Nine felt that they could complete desired tasks with the MPL if allowed additional time to practice beyond the allotted study period. Most study participants (n = 6) reported that the MPL was heavier than their existing prostheses, decreasing their perceived comfort. The favorite user-identified MPL feature was the ability to complete multiple grasps and articulate individual digits. Additional recommendations for MPL improvement included reduced device weight (n = 4) and access to pause/speed features on the physical device (n = 3).
Six individuals reported that awareness of their phantom limb impacted their performance. Seven of 10 participants imagined moving their phantom limb—without the use of physical visualization aids such as a mirror box—in order to operate the pattern recognition control platform. During sessions in which participants felt as if their phantom was restricted to specific positions (i.e. closed hand), they endorsed greater difficulty in changing grasps and controlling the prosthesis. Neither participant with congenital limb absence reported the presence of a phantom limb or relied upon phantom limb imagery for motion visualization. Participants (n = 4) who reported practicing movement visualization (i.e. different hand grasps and opening the hand) at home without the use of a prosthesis subjectively reported greater ease in controlling the MPL during the next session, though this effect was not reliably and quantifiably demonstrated.
This study is the first clinical evaluation of the MPL in individuals with different levels of UE loss. The MPL seeks to restore the strength, utility, dexterity, and intuitive movement of the human arm and hand to individuals with UE loss. Through modular robotic design, the MPL can be adjusted for any level of amputation and is operated via intuitive, non-invasive, pattern recognition control. All participants improved in MPL control without a plateau in functional and TAC assessment scores. Participants significantly increased their number of executable motions and tasks with the MPL over time. All participants with a home prosthesis manipulated a greater number of motions with the MPL than with their conventional prostheses. Individuals with congenital limb absence were also able to control the MPL using this pattern recognition control paradigm, suggesting that there exist cortical motor mechanisms in the absence of limb development that can be trained for prosthetic control.
Participants expressed significant interest in performing activities with the MPL that they had not been able to achieve since the loss of their limb or birth, in the case of congenital participants. The ADLs participants chose to practice during clinical sessions ranged from practical tasks that may facilitate greater independence such as food preparation and the manipulation of small and common objects, long-held goals—one congenital participant desired to write her name with the hand she had been born without—and a return to activities they had previously enjoyed, such as playing catch or mastering the trigger pose to shoot a gun, in the case of a retired Service member. Two participants expressed fervent interest in re-learning to button shirts and tie shoes. These participants believed that their wardrobe reflected their inability to dress as they desired due to functional limitation, as the acts of buttoning and tying are dependent on coordinated individual digit control not offered by other, prior prostheses. Despite practicing these chosen activities during clinical sessions with the MPL, no significant differences in self-reported functional restriction and the ability to perform standardized ADLs were observed across participants in the qualitative instruments administered. Coupled with the recommendation of the majority of participants that additional time be provided for training with the MPL, it is possible that more time with the prosthesis or more detailed qualitative metrics are needed in order to achieve or capture significant objective and self-perceived functional improvements, respectively.
Although participants with trans-humeral amputation controlled a greater number of motion classes and achieved higher normalized TAC motion completion scores than those with more distal amputations, these differences were not statistically significant. Our observations suggest that the MPL may improve function regardless of amputation level, presence of OI/TMR, and prior prosthetic experience.
MPL_19 had worked with the MPL for 80 h in conference demonstrations prior to this study. This participant underwent trans-humeral amputation secondary to malignancy. The ability to generate mental representations of action may be impaired by residual limb and/or prosthesis disuse 34 , 35 . Lengthy time gaps in usage may have contributed to regressions in pattern recognition accuracy due to the practice-dependent strength of motor imagery for prosthetic manipulation. No significant differences were observed with respect to MPL_19′s scores and those of the other participants at this level of limb loss.
This study had several limitations, the most notable of which is study size. Study recruitment was hindered by a paucity of both civilian and military patients with UE loss who met study criteria. However, studies have suggested that issues with prosthetic usability can be detected with as few as four to five subjects 36 . In addition, the short duration of the clinical training period was referenced as a limitation upon participants’ ability to achieve his or her full functional potential with the MPL. Participants specifically requested additional time to practice with the VIE and/or MPL at home in order to continue improving in their ability to execute and switch between motion classes and control the physical prosthesis. Two participants likened this suggestion to their practiced visualization of motion classes in Phases I and II during personal downtime at home, such as during commercial breaks or in bed. This served as motivation for the creation of Phase III, through which we will evaluate MPL use in the home setting over 1 year.
The MPL constitutes an advanced UE prosthetic system capable of restoring intuitive dexterous function for individuals with varied levels and mechanisms of UE loss, from blast injuries to congenital limb absence. Participants controlled more motions with the MPL than with their home prostheses after a short training period and continuously improved in pattern recognition accuracy and functional ability.
Supplementary Information
Acknowledgements.
The authors would like to acknowledge the contributions of Dave Beachler, Josef Butkus, and Annemarie Orr in recruiting participants, socket fabrication, and scoring assessments. The research team would also like to thank Luke Osborn and Matthew Fifer for their help with data analysis and study image and video procurement and approval. This work would not have been possible without the Center for Rehabilitation Sciences Research through the Henry M. Jackson Foundation. We would also like to thank the participants for generously donating their time and efforts to this study.
Author contributions
B.N.P., J.W.T., P.F.P., C.W.M., and R.S.A. contributed to study conceptualization, methodology, and funding acquisition. K.E.Y., B.N.P., A.H., L.S., C.W.M., R.S.A., J.V., and P.F.P. contributed to data collection and study administration. K.E.Y., B.N.P., A.H., C.W.M., R.S.A., J.W.T., and P.F.P. contributed to data analysis. C.W.M., R.S.A., and M.S.J. contributed to device and software design, maintenance, and repair. C.W.M., R.S.A., and P.F.P. supervised all study procedures. K.E.Y., A.H., B.N.P., J.W.T., and P.F.P. contributed to the writing of the original draft. K.E.Y., B.N.P., C.W.M., R.S.A., J.W.T., and P.F.P. contributed to manuscript revision, editing, and figure and table compilation. All authors approved the final version of this manuscript.
Competing interests
The authors declare no competing interests.
Publisher's note
Springer Nature remains neutral with regard to jurisdictional claims in published maps and institutional affiliations.
The online version contains supplementary material available at 10.1038/s41598-020-79581-8.
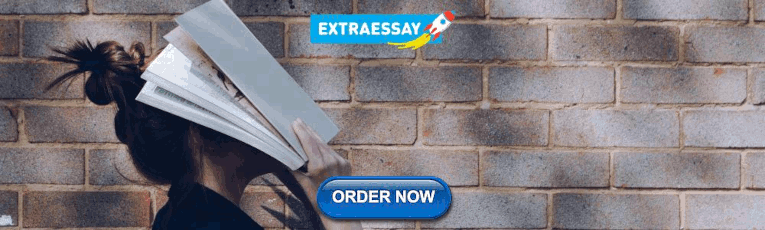
IMAGES
VIDEO
COMMENTS
The incidence of the different levels of upper limb loss is also shown in Figure 2: 16% trans-humeral, 12% transradial, 2% forequarter, 3% shoulder disarticulation, 1% elbow disarticulation, 2% wrist disarticulation, 61% transcarpal, and 3% bilateral limb loss. Traumatism is the first cause of upper limb amputation, predominantly for males 2.
Literature Review on Needs of Upper Limb Prosthesis Users Front Neurosci. 2016 May 12:10:209. doi: 10.3389/fnins.2016.00209. eCollection 2016. Authors Francesca Cordella 1 ... This paper presents a literature review on needs analysis of upper limb prosthesis users, and points out the main critical aspects of the current prosthetic solutions, in ...
using a water tap, combing one' s hair and blowing one' s nose, that. typically unilateral users perform with the intact limb. This work contributes to review the literature on the needs of ...
Literature Review on Needs of Upper Limb Prosthesis Users. REVIEW. published: 12 May 2016 doi: 10.3389/fnins.2016.00209. Edited by: Cuntai Guan, Institute for Infocomm Research, Singapore ...
Hand loss can be perceived Davalli A, Cutti AG, Guglielmelli E and Zollo L (2016) Literature Review on as a devastating damage since it affects the level of autonomy, limiting the capability of performing Needs of Upper Limb Prosthesis working, social, and daily living activities (ADLs).
DOI: 10.3389/fnins.2016.00209 Corpus ID: 6679204; Literature Review on Needs of Upper Limb Prosthesis Users @article{Cordella2016LiteratureRO, title={Literature Review on Needs of Upper Limb Prosthesis Users}, author={Francesca Cordella and Anna Lisa Ciancio and Rinaldo Sacchetti and Angelo Davalli and Andrea Giovanni Cutti and Eugenio Guglielmelli and Loredana Zollo}, journal={Frontiers in ...
Literature review on needs of upper limb prosthesis users. Cordella F; Ciancio A; Sacchetti R; et al. See more; Frontiers in Neuroscience. DOI: 10.3389/fnins.2016.00209. 453 Citations. ... This paper presents a literature review on needs analysis of upper limb prosthesis users, and points out the main critical aspects of the current prosthetic ...
This review presents the state-of-the-art in technologies and materials of the upper limb prosthetics (ULP) for user suitability and needs analyses. The review is critical because availability and use of better performing and thus suitable ULP will not only lower the rate of abandonment of the device but will lead to improvement in the quality ...
Needs of upper limb prosthesis users differ per person, as has also been noted by Carey et al. (2015), who performed a review on the differences between myoelectric and body-powered prostheses . In their review the evidence was insufficient to conclude that the myoelectric or body-powered prostheses offered general advantages over the other.
Henceforth, this review highlights and assesses the effects of hand- prosthetics advancements on the quality of life of patients with/requiring joint replacements. Methods: Relevant literature ...
This work contributes to review the literature on the needs of upper limb prosthetic users and extracts the main requirements to be satisfied by the prosthetic systems in order to improve their acceptability and usefulness. ... Davalli A, Cutti AG, Guglielmelli E and Zollo L (2016) Literature Review on Needs of Upper Limb Prosthesis Users ...
However, the traditional prostheses available on the market are not able to satisfy the users' needs, since they comprise a simplified mechanical construction and do not replace the motor and sensory abilities of the amputated limb. Therefore, with this prostheses, the control and measurement of force is limited, as well as the precise ...
In recent years, researchers and clinicians have become increasingly interested in functional outcomes among users of upper limb prostheses. Evaluations of these outcomes have been performed from different perspectives, including prosthetic management and usage, 1-3 prosthesis satisfaction, stump pain and prosthesis use in different activities 4-7 and effectiveness of prosthetic training ...
As a result of this, further research is needed to fully understand whether biomechanical energy could be harvested for an upper limb prosthetic device. 6. Conclusions. This review presents the state-of-the-art in technologies and materials of the upper limb prosthetics (ULP) for user suitability and needs analyses.
Cordella F, et al. Literature review on needs of upper limb prosthesis users. Front Neurosci. 2016;10:209. Article PubMed PubMed Central Google Scholar Dickstein R, Deutsch JE. Motor imagery in physical therapist practice. Phys Ther. 2007;87(7):942-53. Article PubMed Google Scholar
We present findings of a co-creation project that gained user perspectives from a user survey, and a subsequent workshop involving: people who use an upper limb prosthesis and/or have experienced care services (users), academics, industry experts, charity executives, and clinicians. The survey invited users to prioritise six themes, which ...
Users of prosthetic limbs also conveyed the need for an increase in the range of motion and movement speed of the wrist, ... F. et al. Literature review on needs of upper limb prosthesis users.
Abstract. The choice of a myoelectric or body-powered upper-limb prosthesis can be determined using factors including control, function, feedback, cosmesis, and rejection. Although body-powered and myoelectric control strategies offer unique functions, many prosthesis users must choose one. A systematic review was conducted to determine ...
Even though various treatment options are available in upper limb prosthetics, high rejection rates indicate that current prosthetic devices meet the needs of users only partially or not at all [2, 3]. Therefore, users and professionals should be included in the research and development process for new prostheses.
The results of these investigations may be used to inform the development of future prosthetic technologies that are appealing to individuals with upper limb loss. BackgroundNovel techniques for the control of upper limb prostheses may allow users to operate more complex prostheses than those that are currently available. Because many of these techniques are surgically invasive, it is ...
Background High rejection rates of upper limb prosthetics indicate that current prosthetic devices only partially meet user demands. This study therefore investigated the benefits and challenges with current prostheses, associated services and potential areas for improvement from the perspective of upper limb prosthesis users and various professionals working in the field of upper limb and ...
A review of technology, materials and R&D challenges of upper limb prosthesis for improved user suitability. R. Brack E. Amalu. Materials Science, Medicine. Journal of orthopaedics. 2021; 16. PDF. ... A literature review on needs analysis of upper limb prosthesis users is presented, and the main critical aspects of the current prosthetic ...
Upper limb prostheses can be divided into passive and active devices. Conventional body-powered prostheses offer simplicity, durability, lightness of weight, and tension feedback to the user with control of basic hand grasps 3 .