If you're seeing this message, it means we're having trouble loading external resources on our website.
If you're behind a web filter, please make sure that the domains *.kastatic.org and *.kasandbox.org are unblocked.
To log in and use all the features of Khan Academy, please enable JavaScript in your browser.
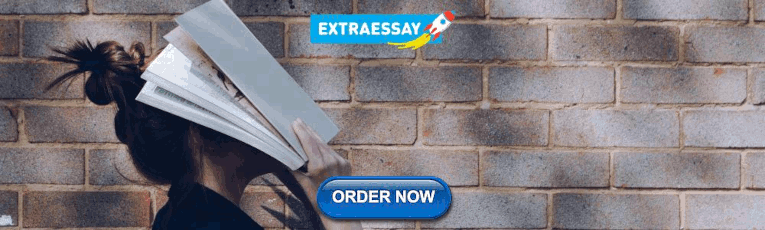
Unit 4: Chemical bonding and molecular structure
About this unit.
Why do atoms combine? Why are only certain combinations possible? Why do molecules possess definite shapes? To answer such questions different theories and concepts have been put forward from time to time.
In this fourth unit of class 11 chemistry, we can answer the above questions by learning Kössel-Lewis approach, Valence Shell Electron Pair Repulsion (VSEPR) Theory, Valence Bond (VB) Theory and Molecular Orbital (MO) Theory.
Kossel-Lewis approach to chemical bond
- Ionic bonds (Opens a modal)
- Ionic bonds: Reaction of metals & Non-metals (Opens a modal)
- Covalent bonds (Opens a modal)
- Single and multiple covalent bonds (Opens a modal)
- Metallic bonds (Opens a modal)
- Drawing Lewis diagrams (Opens a modal)
- Predicting bond type (metals vs. nonmetals) (Opens a modal)
- Worked example: Lewis diagram of formaldehyde (CH₂O) (Opens a modal)
- Worked example: Lewis diagram of the cyanide ion (CN⁻) (Opens a modal)
- Exceptions to the octet rule (Opens a modal)
- Worked example: Lewis diagram of xenon difluoride (XeF₂) (Opens a modal)
- Lewis diagrams 4 questions Practice
Bond energies
- Bond length and bond energy (Opens a modal)
- Worked example: Interpreting potential energy curves of diatomic molecules (Opens a modal)
- Lattice energy (Opens a modal)
- Intramolecular force and potential energy 4 questions Practice
Bond parameters
- Resonance (Opens a modal)
- Formal charge (Opens a modal)
- Worked example: Using formal charges to evaluate nonequivalent resonance structures (Opens a modal)
- Resonance and formal charge 4 questions Practice
Polarity of bonds
- Molecular polarity (Opens a modal)
- Electronegativity and bonding (Opens a modal)
- Predicting bond type (electronegativity) (Opens a modal)
- Types of chemical bonds 4 questions Practice
VSEPR theory
- VSEPR for 2 electron clouds (Opens a modal)
- VSEPR for 3 electron clouds (Opens a modal)
- More on the dot structure for sulfur dioxide (Opens a modal)
- VSEPR for 4 electron clouds (Opens a modal)
- VSEPR for 5 electron clouds (part 1) (Opens a modal)
- VSEPR for 5 electron clouds (part 2) (Opens a modal)
- VSEPR for 6 electron clouds (Opens a modal)
- VSEPR 4 questions Practice
- VSEPR - II 4 questions Practice
Hybridisation
- sp³ hybridization (Opens a modal)
- Steric number (Opens a modal)
- sp² hybridization (Opens a modal)
- sp hybridization (Opens a modal)
- Worked examples: Finding the hybridization of atoms in organic molecules (Opens a modal)
- Bond hybridization 4 questions Practice
Molecular Orbital Theory
- No videos or articles available in this lesson
- Molecular orbital theory 4 questions Practice
- Applications of Molecular Orbital Theory 4 questions Practice
Hydrogen bonding
- Hydrogen bonding in water (Opens a modal)
- Hydrogen bonding (Opens a modal)
- Water and hydrogen bonding 4 questions Practice

An official website of the United States government
The .gov means it’s official. Federal government websites often end in .gov or .mil. Before sharing sensitive information, make sure you’re on a federal government site.
The site is secure. The https:// ensures that you are connecting to the official website and that any information you provide is encrypted and transmitted securely.
- Publications
- Account settings
Preview improvements coming to the PMC website in October 2024. Learn More or Try it out now .
- Advanced Search
- Journal List

Chemical Bonding: The Journey from Miniature Hooks to Density Functional Theory
Our modern understanding of chemistry is predicated upon bonding interactions between atoms and ions resulting in the assembly of all of the forms of matter that we encounter in our daily life. It was not always so. This review article traces the development of our understanding of bonding from prehistory, through the debates in the 19th century C.E. bearing on valence, to modern quantum chemical models and beyond.
1. Introduction
Bonding is what separates chemistry from physics. If the understanding of atoms and their component particles belongs primarily to the realm of physics, then chemistry is concerned with the aggregation of atoms into chemical entities held together by bonds . If science is a language, and atoms are the letters, bonding is the mechanism by which the letters are combined into words. The International Union of Pure and Applied Chemistry (IUPAC) states that “there is a chemical bond between two atoms or groups of atoms in the case that the forces acting between them are such as to lead to the formation of an aggregate with sufficient stability to make it convenient for the chemist to consider it as an independent ‘molecular species’” [ 1 ].
Although the concept of bonding is inherent in the discipline of chemistry and its distinction from its neighbor, physics, our modern understanding of bonding is relatively recent. In the 19th century C.E., as chemists were making enormous advances in the methods for the preparation, purification, and characterization of new molecular species, they were in parallel struggling with developing models for understanding the structure and constitution of these compounds. However, the ideas and concepts did not spring fully-formed into the minds of the 19th century scientists, but can rather be traced as far back as when mankind started to think about matter and its components. This article attempts to give an overview of how we made this journey of discovery and will attempt to demonstrate how the concepts that are today so fundamental that we do not even think about them, proved troublesome and time-consuming to our forebears.
This article is concerned primarily about the roots and origins of our understanding of bonding. Accordingly, it concentrates upon the introduction of new ideas and the controversies and changes that resulted rather than providing a full history of their development after acceptance. The reader is referred to modern texts on bonding [ 2 , 3 , 4 , 5 ], in particular the three-volume work by Mike Mingos celebrating the centennial of the chemical bond [ 6 , 7 , 8 ]. As always, we are indebted to those historians of science who have trodden this path before us in such an able and comprehensive manner [ 9 , 10 , 11 , 12 , 13 ]. A final note concerns the abbreviations of journals; over the years, many journals have changed their titles, some with a confusing and monotonous regularity. For consistency, the CASSI (CAS Source Index) abbreviations are used throughout. In those cases where a journal has changed title, the primary abbreviation is used, even though the original journal title may differ slightly. As an example, the abbreviation Ann. Phys. (Berlin, Ger.) is used for the entire period 1819 to date, even though the contemporary title varied amongst Annalen der Physik und Physikalischen Chemie , Annalen der Physik und Chemie , Drude’s Annalen , Poggendorff’s Annalen der Physik , Annalen der Physik (Leipzig, Germany) or Annalen der Physik (Weinheim, Germany) ; similarly, the abbreviation Justus Liebigs Ann. Chem. is used for Justus Liebigs Annalen der Chemie , Annalen der Chemie und Pharmacie and Justus Liebigs Annalen der Chemie und Pharmacie . After that bibliometric aside, let the journey begin!
2. Early Ideas up to the Age of Reason
This section looks at the genesis of atomism and investigates the then contemporary ideas regarding the interactions between atoms that resulted in matter possessing distinct and unique properties. The primary focus of this review is on bonding, but to comprehend how modern views of bonding evolved, we need a broad overview of how our understanding of atoms, which are ultimately the objects that are connected by the bonds, developed. It is convenient and conventional to start this discussion with the philosophies developed by the Early Greek civilization, although this approach is neither chronologically nor historically strictly correct.
2.1. The Greeks Had a Word for It
The word atom (and related terms such as atomic, atomistic, and atomism) is derived from the Greek word: ἄτομος (atomos) meaning indivisible or uncuttable. Most histories of atomism begin with the Greek philosopher Leucippus (Λεύκιππος, Leúkippos) who was supposed to have lived sometime in the fifth Century B.C.E. Very little is known about Leucippus who was credited by Aristotle and Theophrastus as the originator of atomism, although a subsequent champion of the philosophy, Epicurus, maintained that Leucippus was not a historical figure. There is little historical material relating to the philosophy of Leucippus, and his role in the development of atomism is fused irrevocably with the name of his pupil Democritus (Δημόκριτος, ca. 460–ca. 370 B.C.E., Figure 1 ) [ 14 ]. The atomistic philosophy was subsequently refined and extended by Epicurus (Ἐπίκουρος, 341–270 B.C.E.) and Lucretius (Titus Lucretius Carus, ca. 99–ca. 55 B.C.E.). Although only fragments of work that can be ascribed to Leucippus and Democritus survive [ 15 , 16 , 17 , 18 , 19 ], Lucretius’ poem De rerum natura (On the Nature of Things) still exists and is a robust didactic defense of atomism [ 20 ].

Democritus, together with his mentor Leucippus, are usually credited with the introduction of the atomistic model to Greek philosophy. (Image source, Public Domain, http://www.phil-fak.uni-duesseldorf.de/philo/galerie/antike/demokrit.html ).
The atomistic philosophy held that the universe was composed of atoms and voids and that matter was composed of these indivisible atoms as building blocks. The void was empty space between the atoms [ 21 ]. The fundamental thesis was that if you sub-divided an object a sufficient number of times, you would eventually reach a particle that could not be further divided. These atoms were of different types, weights, and shapes, and some possessed hooks or spikes, allowing them to be combined in different ways to generate various types of matter. This is the first physical model that we encounter for chemical bonding. Note that the term atom does not correspond to modern usage; for example, cooking salt (NaCl), stone or wood, was regarded as being composed of atoms of salt, stone, or wood, respectively. Implicit in the atomistic philosophy was the assumption that atoms could be created or destroyed. As Lucretius wrote in De rerum natura .
“Two kinds of bodies are to be distinguished: there are primary elements [atoms] of things, and objects compounded of primary elements [atoms]. As for the primary elements, no force has power to extinguish them … The ultimate particles are solid and contain no void … They must of necessity be everlasting.” [ 22 ].
The properties of matter were related to the nature of the atoms and their bonding. Hard matter such as metal, stone or diamond is composed of atoms mutually hooked or stuck together in an extended array. Liquids are composed of smooth and spherical atoms, with higher viscosities being related to rougher surfaces or more hooks between the atoms. It must be remembered that early Greek science was not an experimental science as we would recognize, but rather a philosophical construction. There was no question of performing experiments to probe the validity of the atomistic model.
The atomistic philosophy was not generally accepted and fell into disrepute in favor of an alternative, originally formulated by Thales (Θαλῆς, ca. 624–ca. 548 B.C.E.). He postulated that all matter was simply a different manifestation of a single basic element, which he subsequently decided was water. The description element does not equate scientifically to our modern understanding of the word, although the concept of a fundamental building block is similar. The philosophy was modified by Anaximenes of Miletus (Ἀναξιμένης ὁ Μιλήσιος; ca. 586–ca. 526 B.C.E.), who suggested that air, not water, was the basic element in an attempt to avoid the need to postulate the existence of a vacuum between material objects, particularly between the Earth and the heavens. In his philosophy, water was a condensed form of air. A third proposal came from Heraclitus of Ephesus (Ἡράκλειτος ὁ Ἐφέσιος, ca. 535–ca. 475 B.C.E.), who suggested that matter was characterized by change and that the basic element was neither water nor air, but fire. It took Empedocles (Ἐμπεδοκλῆς, ca. 494–ca. 434 B.C.E.) to question whether it was necessary to postulate just a single basic element and he developed a philosophy in which matter was composed of the three elements water, air, and fire together with a fourth, earth, that he had added. This is the doctrine of the four elements that we today associate with the philosopher Aristotle (Ἀριστοτέλης, ca. 384–ca. 322 B.C.E., Figure 2 ). Aristotle also associated the elements with the properties of matter in a philosophy that had a remarkable internal consistency. However, not to be outdone, Aristotle also added a fifth element, ether, which was found in the heavens and is the perfect form of the other four “imperfect” elements. The Aristotelian philosophy is best embodied in the work of Plato (Πλάτων, ca. 428–348 B.C.E.), who equated the shapes of the elements fire, earth, air, and water with those of the “platonic solids”, the tetrahedron, cube, octahedron, and icosahedron. The closest that the Aristotelian model comes to bonding is the rather complex concept of reciprocation [ 16 , 23 , 24 ].

Bust of Aristotle. Marble, Roman copy after a Greek bronze original by Lysippos from 330 BC; the alabaster mantle is a modern addition (Image source, Public Domain, https://commons.wikimedia.org/wiki/File:Aristotle_Altemps_Inv8575.jpg ).
Aristotle dogmatically rejected the concept that there was any limit to the sub-division of matter, partly because it implied the existence of a vacuum between atoms and in so-doing, essentially condemned the atomistic model to obscurity. The last serious discussions of early atomism that have survived to modern times are attributed to Galen (129–216 C.E.) [ 25 ]. Paradoxically, interest in the Aristotelian philosophy in medieval Europe resulted in a rediscovery and revival of the atomistic ideas.
2.2. Atomism in Other Early Cultures
Although the emphasis of this review is on bonding rather than atomism, bonding cannot exist without an atomic description and it is interesting to make a short deviation to see how, as so often happens in science, parallel ideas developed in multiple cultures. It seems likely that the ideas of atomism, which are today presented as Greek rationalism were predated by philosophies developed in India and the Middle East.
2.2.1. Indian Atomism
In the eighth century B.C.E., the Vedic sage Aruni (also known as Uddalaka or Uddalaka Aruni), stated that “particles too small to be seen mass together into the substances” [ 26 ]. This implies a limit to sub-division of matter to give primal particles and can be seen as the beginning of atomism in the Hindu tradition. Sometime between the sixth and second centuries B.C.E. the Maharishi Kanad (कणाद) proposed an atomistic model of the universe in his book Vaisheshika Sutra which was the origin of the Vaisesika philosophy. The philosophy is complex but resembles the later Greek atomism in postulating that matter can only be sub-divided until a smallest entity (anu or paramanu) is reached. The paramanu are indivisible and indestructible. By the seventh century B.C.E. three distinct atomistic schools (the Charvaka, the Jain and the Ajivika) had evolved. Subsequently, the Nyaya and Vaisesika philosophy, developed a model of the universe based upon four basic types of element, reminiscent of the Aristotelian philosophy, but considerably more sophisticated in the relation of the atoms to the properties associated with them. The Nyaya, Vaisesika, and Jaina schools developed bonding models to rationalize the ways in which atoms combined into more complex matter, based upon the formation of dyads and triads [ 27 , 28 ]. This tradition continued well into the modern era with texts as late as the 12th century C.E. discussing atoms made of energy as being point-sized and indestructible [ 29 , 30 , 31 ].
In parallel to the development of the Hindu atomist philosophy, the Buddhist tradition was developing a world view close to the Greek [ 32 , 33 ]. In the earliest Buddhist tradition, atoms were not regarded as permanent, but by the seventh century C.E., the philosophy had evolved to one of indestructible atoms. The Buddhist philosophy does not seem to have had a well-developed model for bonding between atoms.
2.2.2. Chinese Atomism
It is, perhaps, surprising that China did not develop an atomistic philosophy. Nevertheless, and despite regular cultural, philosophical, and commercial exchange with the Indian sub-continent as well as Buddhist scholars, an atomistic model was not established in the indigenous Chinese natural philosophy. Needham identified fleeting examples of atomistic ideas, but these were never adopted into the mainstream and remained, at best, ephemeral [ 34 ]. One can only speculate as to the models and descriptions of bonding that might have been developed.
2.2.3. Islamic Atomism
There is no doubt that the early Islamic philosophers were aware of the atomistic philosophies. At the same time as Buddhist philosophies were being formulated in the 12th century C.E., Islam al-Ghazali (1058–1111 C.E.) was developing the Asharite school predicated upon the postulate that atoms were the only permanent material objects [ 35 ]. The Islamic philosophy developed atomism as a consequence of the need for substance to occupy space ( tahayyuz ) and atoms were regarded as indestructible. Convincing arguments have been presented that the Islamic atomistic culture derives from the work of Galen [ 36 ]. The Islamic tradition paralleled the ancient Greek, and the atomistic model was rejected by Ibn Rushd (أبو الوليد محمد ابن احمد ابن رشد, Latinized as Averroes, 1126–1198) in favor of a model close to the Aristotelian [ 37 ].
2.3. The Revival of Atomism in Europe—Entering the Age of Reason
After the decline and eventual fall of the Roman empire, Europe entered a period in which intellectual achievement was less recognized and for which the historical record is lacking. By the beginning of the second millennium C.E., Europe was beginning to look outwards and intellectualize again, in particular through interactions with the Middle East (unfortunately partially typified by the Crusades) and trading of goods along the Silk Road. In addition to the military and commercial exchanges, Europeans were also exposed to new cultures and rediscovered their own forgotten cultural heritage [ 38 ].
The first wave of this rediscovery can be dated to the period ca. 1250–1350 C.E., when the works of Aristotle were translated from Greek or Arabic into Latin, the scientific language of the day. Paradoxically, the Aristotelian arguments against the atomistic philosophy served to reignite interest in this model in Europe. The implication that matter could be transformed between various forms appealed to both the venal and the scientific nature of man and spawned the mix of mythology and science that we categorize as alchemy. By the 14th century C.E., European scholars had rediscovered the work of Lucretius, in particular De rerum natura ( Figure 3 ) and by the 15th century C.E. printed copies had become available to study.

The title page of De rerum natura by Lucretius, from the 1675 edition by Tanaquil Faber. (Image source, Public Domain, https://upload.wikimedia.org/wikipedia/commons/6/6e/Lucretius_De_Rerum_Natura_1675_page_1.jpg ).
2.3.1. Early Conflicts with Christian Orthodoxy
Lucretius was one of the leading Roman advocates of the philosophy known as Epicureanism (after Epicurus, Ἐπίκουρος, Latinized Epíkouros, 341–270 B.C.E.), which held an atomistic model of the world at its core [ 39 , 40 , 41 ]. The establishment of Christianity as a Roman state religion by the Emperor Constantine in 313 C.E. resulted in the suppression of Epicureanism (together with its associated atomistic theories), mainly because the philosophy emphasized the neutrality of the gods and their non-interference in the affairs of man. In the early part of the second millennium C.E., atomism remained associated with Epicureanism and in the strongly Christian establishment of the time, atomic models for matter were not intensively studied in Europe.
Galileo Galilei (1564–1642 C.E.) was one of the early scientists who considered atomistic views of matter. Although not directly related to his atomistic views, Galileo had his own differences with the established Christian church regarding his astronomical observations and conclusions [ 42 ]. In his 1612 monograph Discorso al Serenissimo Don Cosimo II¿Intorno alle cose, che Stanno in su l¿acqua, o che in quella si muovono (A Discourse Presented to the Most Serene Don Cosimo II Great Duke of Tuscany: Concerning The Natation of Bodies Upon, or Submersion In, the Water), Galileo uses an atomistic model to describe the interactions between objects and water [ 43 , 44 , 45 ]. By 1623, Galileo had further developed his observations into the more coherent atomist form in his publication Il Saggiatore (The Assayer), although the philosophy belongs more generally to the school of corpuscularianism, which is atomistic but allows the subdivision of atoms. Certain statements place it specifically in the atomistic tradition rather than that of corpuscularianism, “and perhaps when such attrition stops at or is confined to the smallest quanta, their motion is temporal and their action calorific only; but when their ultimate and highest resolution into truly indivisible atoms is arrived at, light is created” [ 46 , 47 ].
2.3.2. Reconciling Church and Atomism
Pierre Gassendi (1592–1655 C.E.) was a philosopher and scientist who both revived the Epicurean atomistic philosophy and attempted to reconcile it with the Christian church [ 48 , 49 ]. The most complete exposition of the Epicurean atomist view is given in his Syntagma philosophiae Epicuri (The Structure of Epicurean Philosophy) published in 1649 [ 50 ]. An important feature of the Epicurean atomism was the concept that atoms had “angles and hooks” which allowed them to interact with one another, surely the prototypical description of bonding. One of the earliest Christian refutations of Epicureanism dates to Lucius Caecilius Firmianus Lactantius (c. 250–c. 325 C.E.), who inter alia disputed that atoms could have angles and hooks [ 51 ]. Gassendi provided a detailed answer to all of Lactantius’ arguments in a robust defense of atomism. Although Gassendi might have been the atomist par example at the beginning of the 17th century C.E., he does not appear to have significantly predated John Dalton in giving a modern description of atoms. In his compositionality thesis, Gassendi suggested that combinations of different atoms could assemble into molecules (although this word was not yet in general use for such assemblies), thus defining their macroscopic properties. However, he does not appear to have made the next logical step of associating molecular diversity with the atoms in the combination. Gassendi comes close to developing an atomic or molecular level description that implies affinity (if not bonding) in his discussions of solubility. He explained that aqua regia (HNO 3 and HCl) dissolves gold, and aqua fortis (HNO 3 ) dissolves silver because the gold atoms fit into the holes between atoms of aqua regia and the silver atoms fit into the holes between atoms of aqua fortis . One of his greatest contributions was the reconciliation of the atomist view with the orthodox Christian view, perhaps through sophistry, by arguing that everything in the universe, including the atoms, were created by God. Gassendi does not talk about molecules directly, but his description of “seeds of things” ( semina rerum ) equates with Boyle’s molecula . Outside the scientific arena, Gassendi is best remembered today for his disputes with his contemporary, the philosopher René Descartes.
It would be disingenuous to attempt to describe the philosophical contributions of Descartes in a few lines and we are here only concerned with his atomistic views. Many of the views presented by Descartes in his Principles of Philosophy appear to the modern reader to be very close to those of the Greek atomists [ 52 , 53 ]. There is, however, an important distinction: Descartes believed that matter could, in principle, be infinitely sub-divided. The reflection that this division in principle could (only) be achieved by God provides another point of reconciliation with Christian theology. The bulk of the philosophy is phenomenologically similar to atomism, with the exception that he talks of corpuscles (rather than atoms), which are the basic units that matter is assembled from. This philosophy is also known as corpuscularianism [ 54 , 55 ]. In his views on human perception of matter, Descartes is very close to modern molecular pharmacology, proposing that sensations such as taste are results of the shapes and sizes of the corpuscles or their assemblies. However, for our main topic of bonding, Descartes makes no great contribution as he explicitly rejects bonds between the particles of matter to explain the bulk physical properties. Nevertheless, he does recognize that there must be interactions between corpuscles.
2.3.3. Anti-Aristotleanism and an Early Case for Atomism
As so often happens in science, the time was right for innovation and new ideas. Insomuch as innovation also involves elements of iconoclasm, the views of the influential anti-Aristotelian thinkers are relevant. This school rejected the Aristotelian philosophy in favor of an Epicurean one founded on atomistic thought. This is not the place to examine the conflict that arose regarding these views within the established church. Nevertheless, one particularly influential work published in 1621 is Philosophiae Naturalis adversus Aristotelem libri XII In quibus abstrusa Veterum Physiologia restauratur et Aristotelis errores solidis rationibus refelluntur a Sebastiano Bassone by the eponymous Sébastien Basson (1573?–?) [ 56 ]. This work systematically demolishes the Aristotelian world view and expounds the atomistic one. However, Basson does not embrace a model in which compounds with different properties are assembled from component atoms, as seen in the work of Sennert (see Section 2.4.1 ).
2.3.4. An Aside on the Word Molecule
In the literature of the 18th century C.E., the words atom and molecule are used with varying degrees of precision. Many authors, including John Dalton, use the term atom to also refer to the smallest unit of a molecular species (for example, atoms of water). At the same time, the word molecule was beginning to be used for discrete species composed of atoms (in the modern sense). Most sources trace the etymology to the French word molécule , which in turn is derived from the New Latin molecula which is purported to be a diminutive of the Latin moles , meaning a mass. One of the earliest uses of molecula in a sense that modern readers would understand dates to January 1666 in correspondence between Robert Boyle and D. Coxe, “These Subtilized principles meeting together may bee readily united: which Substances thus united Constitute a little masse, or molecula of mettall, many of which are usually associated before they appeare in a visible or sensible forme” [ 57 ]. Interestingly, Boyle does not use the word in his Sceptical Chymist of 1661 ( Figure 4 , vide infra ).

The title page of the 1661 book The Sceptical Chymist by Robert Boyle, a pioneering work in the history of chemistry that questioned the Greek notions of elements and Aristotelian atomism (Image source, Public Domain, http://www.gutenberg.org/files/22914/22914-h/22914-h.htm ).
2.4. Early Ideas in the Scientific Age
The transition into the scientific age is a rather arbitrary boundary that we have made on the basis of individuals who the modern mind perceives of as scientists rather than philosophers or alchemists— mea culpa . We have also now restricted the discussion to those atomists who explicitly made consideration of chemical bonding and interactions between the atoms. In general, no distinction will be made between atomists and corpuscularianists.
One of the early scientific converts to atomism (or at least to some of its aspects) was Francis Bacon, a philosopher, politician, statesman, and scientist. In his 1612 work De Principiis atque Originibus he considers and rejects the alchemical ideas of transformation and argues for atomism or corpuscularianism [ 58 , 59 , 60 ]. However, in later work, Bacon argued that the bulk properties of matter arose from the size and motion of corpuscles rather than individual atoms and eventually rejected the doctrine of atoms using an Aristotelian argument relating to the absence of a vacuum in nature [ 61 ]. Despite his chemical and alchemical credentials, Bacon does not appear to have speculated significantly on the nature of chemical bonding.
2.4.1. Daniel Sennert and a Rational Atomism
One of the early contributions that identify interactions between atoms comes from Daniel Sennert (1572–1637 C.E.) who published his Hypomnemata Physica in 1636 [ 62 ]. This remarkable document provides an excellent overview of the 17th century C.E. interpretation and incorporation of the Greek ideas into the contemporary philosophy. The debate between atomists and corpuscularianists is moderated by a hierarchical organization of matter. In her excellent assessment of his contributions, Michael [ 63 ] summarizes Sennert’s views on atoms and bonding:
- (1) The fundamental units of matter are extremely minute bodies called atoms, each of which has a specific substantial form.
- (2) The simplest atoms are atoms of the elements. Each is indivisible and immutable.
- (3) There are various grades of atoms, and each higher grade of atom is composed of an organization (or structure) of the next lower grade of atoms.
- (4) Each body that is not an atom is composed of bonded atoms
Sennert’s elements are the Aristotelian big four—earth, air, fire, and water. Atoms of the “modern” elements, compounds and materials are at the higher organizational levels. This is about as good as it is going to get, in the absence of a modern definition of an element. Sennert described the level of organization corresponding to molecules as prima mista and postulated that prima mista could be converted back to atoms (although these may have been the Aristotelian atoms).
2.4.2. Robert Boyle—The End of Alchemy or the Beginning of Chemistry?
For chemists, Robert Boyle (1627–1691 C.E.) is immortalized in Boyle’s law:
which relates the volumes of a gas measured at different pressures at a given temperature. However, he was a very accomplished scientist who made significant contributions to a wide range of chemical and other scientific areas. Although widely acclaimed as “the father of modern chemistry” in standard histories of chemistry [ 10 , 13 ], revisionists correctly identify that he was not alone in the chemical firmament, but disagree about the degree of influence that he had on the development of the subject [ 64 , 65 , 66 ]. Fortunately, it is not our mandate to enter into this debate. Even as a young man, Boyle was a convert to atomistic or corpuscularian views, and an early work dating from 1654 (only published after his death) entitled Atomicall Philosophy is essentially a restating of the views of Gassendi, Descartes, and Sennert [ 67 , 68 ]. However, Boyle’s 1661 publication The Sceptical Chymist : or Chymico-Physical Doubts & Paradoxes [ 69 ] is of relevance to our story. This monograph is written as a discourse on the nature of matter between five protagonists and which Boyle uses to forward the atomist (or rather, corpuscularian) philosophy. The text is rather difficult for the modern reader, who is generally not accustomed to scientific arguments being presented in a discursive style, but this would have been familiar to his contemporary scientists and philosophers. Although Boyle was probably not the originator of the modern definition of an element, he discarded the Aristotelian four elements for a more robust and experimentally verifiable understanding of an element:
“I now mean by Elements, as those Chymists that speak plainest do by their Principles, certain Primitive and Simple, or perfectly unmingled bodies; which not being made of any other bodies, or of one another, are the Ingredients of all those call’d perfectly mixt Bodies are immediately compounded, and into which they are ultimately resolved”.
However, Boyle the experimentalist did not believe that any of the materials known to science at that time were “perfectly unmingled bodies” (elements), although there is a relatively long list of substances known to him that we today call elements, including antimony, arsenic, bismuth, carbon, copper, gold, iron, lead, mercury, silver, sulfur, tin, and zinc. We have already noted that Boyle was an early adopter of the term molecula to describe an assembly of atoms or corpuscles. Boyle proposed that chemical change was associated with rearrangements within or between clusters of corpuscles—a concept that can be correlated with the modern view of chemical change being associated with the making and breaking of bonds. In another of his works, The origine of formes and qualities , Boyle gives a description of the assembly of the corpuscles that resonates remarkably with the modern molecular model [ 70 ]:
“There are also Multitudes of Corpuscles, which are made up of the Coalition of several of the former Minima Naturalia; and whose bulk is so small, and their Adhesion so close and strict, that each of these little Primitive Concretions or Clusters (if I may so call them) of Particles is singly below the discernment of Sense …”
Although Boyle was a chemist from the Age of Reason par excellence , he apparently retained a foot in the alchemical roots of his subject. The debate continues as to where his heart really lay, on the one hand he is seen as the alchemist criticizing some alchemical theories rather than defending a modern view of chemistry [ 71 ] and who went as far as communicating to the Royal Society his conversion of mercury into an “essential mercury”, which would allow transmutation to gold [ 72 ], and on the other he is eulogized as the first true scientist [ 73 ].
2.4.3. Isaac Newton—Another Alchemist?
Sir Isaac Newton addressed the question of attraction between atoms in Query 31 of his work Opticks [ 74 ], and gives a good contemporary view of the ideas concerning the forces between atoms. After writing “The Parts of all homogeneal hard Bodies which fully touch one another, stick together very strongly. And for explaining how this may be, some have invented hooked Atoms, which is begging the Question; and others tell us that Bodies are glued together by rest, that is, by an occult Quality, or rather by nothing; and others, that they stick together by conspiring Motions, that is, by relative rest amongst themselves”, he continues with his own ideas, “I had rather infer from their Cohesion, that their Particles attract one another by some Force, which in immediate Contact is exceeding strong, at small distances performs the chymical Operations above-mention’d, and reaches not far from the Particles with any sensible Effect”. In the text, Newton shows a broad knowledge of chemistry but, like most of his contemporaries, does not always make a clear distinction between physical change and chemical reaction. In many respects the ideas of Newton are closely related to the ideas of chemical affinity and he gives examples of chemical reactions arranged in a similar manner to Geoffroy (see Section 3.1.1 ).
2.4.4. Bryan and William Higgins—A Historical Aside
Another player in our unfolding drama is Bryan Higgins (1737?–1818) who practiced as a physician and published a number of treatises and books on practical chemistry. He was interested in the forces present in (classical) elements and developed a model based upon the repulsion of like atoms in all elements except earth and water [ 75 ]. In this model, he further developed Newton’s ideas of mutual repulsion between atoms (or molecules in modern terms) of gases. He also developed a model for the formation of compounds AB from acids and bases (in his terminology, atoms of A and B) based upon a greater attractive force in AB than the repulsive forces between A and B atoms. This predates the Brönsted acid-base model and anticipates ionic bonding between cations and anions.
William Higgins (1762?–1825) was the nephew of Bryan Higgins and shared the familial interest in the attractive forces between atoms and refined his uncle’s model to consider interactions between more than two particles [ 76 , 77 ]. The similarity of the vector diagrams of the forces that Higgins presents to modern covalent bonds is remarked upon by Partington [ 11 ].
3. The Nineteenth Century C.E.
3.1. valence and affinity.
By the beginning of the 19th century C.E., the scientific world was developing a large enough body of chemical knowledge to begin meaningful discourse on the forces holding atoms together in molecules. One of the critical aspects was the development of a theory to explain and predict how many and what type of atoms could be bonded to each other. The time has come for a discussion of valence or valency.
3.1.1. Chemical Affinity—Driving Force but not Bonding
By the 19th century C.E., the term affinity was understood to refer to the number of, and preference for, the different combinations into which substances could enter and form new compounds. The term had been used earlier with some subtly different meanings [ 78 , 79 ]. One early usage is attributed to Albertus Magnus (1193–1280) who used the word affinitas in the context of chemical reactivity, for example he describes the reaction of sulfur with metals on the basis of a shared affinitas [ 80 , 81 , 82 ]. In general, Magnus expounded the Aristotelian vision and although his use of affinity is very early, it has little further relevance for us. The same applies to contributions on chemical affinity or elective affinity from Francis Bacon, Robert Boyle, John Mayow, Johann Rudolf Glauber, Nicolas Lémery, Isaac Newton, and Georg Ernst Stahl [ 83 ]. In general, the concept of affinity evolved into those of driving force, free energy, and chemical thermodynamics. Nevertheless, it is worth noting that Étienne François Geoffroy (1672–1731) [ 84 , 85 , 86 ] and Torbern Olof Bergman [ 87 , 88 ] both produced “affinity tables”, which ordered substances according to their tendency to react with each other ( Figure 5 ).

Geoffroy’s affinity tables represented one of the early attempt to bring order to the wealth of chemical information [ 84 , 85 , 86 ] (Image source, Public Domain, https://commons.wikimedia.org/wiki/File:Affinity-table.jpg ).
The difficult relationship between chemical affinity and valence was well expressed by Falk in 1914, when he wrote, “The separation of these two problems, valence and chemical affinity, makes it clear that while a great number of substances may be predicted from a consideration of valence structures alone, questions of chemical affinity, or relative stability, limits the number of these substances which are actually known or may be prepared” [ 89 ].
3.1.2. Valence or Valency
For a true understanding of modern chemistry, the concept of valence (or valency) is critical [ 90 , 91 ]. The IUPAC definition, “The maximum number of univalent atoms (originally hydrogen or chlorine atoms) that may combine with an atom of the element under consideration, or with a fragment, or for which an atom of this element can be substituted” is unambiguous and, thus, assigns valences of four and five to carbon in methane CH 4 and methanium CH 5 + , respectively. The principal driver for the acceptance of the modern sense of valence was August Kekulé [ 92 ]. In one of his critical publications, Kekulé wrote “ Die Zahl der mit Einem Atom (eines Elementes, oder wenn man bei zusammengesetzteren Körpern die Betrachtung nicht bis auf die Elemente selbst zuruckfuhren will, eines Radicales) verbundenen Atome anderer Elemente (oder Radicale) ist abhängig von der Basicität oder Verwandtschaftsgrösse der Bestandtheile ” (The number of atoms of other elements (or radicals) connected with an atom (an element, or, if one does not want to reduce the observation to the elements themselves in the case of composite bodies, a radical) depends on the basicity or relationship size of the components) [ 92 ]. Valence was critical as a driver for the understanding of organic chemistry and also the development of the coordination chemistry model proposed by Alfred Werner. Nevertheless, it is a difficult and diffuse concept and Ramsberg comments, “Strictly speaking, valence … was a number that possessed no physical significance” [ 90 ]. The frustration in the 19th century C.E. was tangible: in 1876, Victor Meyer wrote, “ Allein diese Arbeitsfülle und die grosse Zahl der gewonnenen Vortheile haben niemals das Bewusststein unterdrücken können, dass wir über das eigentliche Grundprincip unserer heutigen Anschauungen, über die Nature dessen, was wir eine Valenz oder Verwandtschaftseinheit nennen, vorläufig noch vollkommen im Unklaren sind ” (The sheer volume of work and the large number of advantages gained have never been able to suppress the awareness that we are currently completely unclear about the basic principle of our current views and the nature of what we call valence or affinity) [ 93 ].
The true modern definition of valence originated with Edward Frankland ( Figure 6 ) in 1852 when he observed, “When the formulae of inorganic chemical compounds are considered … it is sufficiently evident … no matter what the character of the uniting atoms may be, the combining power of the attracting element… is always satisfied by the same number of these atoms” [ 94 ]. Frankland’s “combining power” is the first formulation of the concept of valence and was adopted by the German community, being translated variously as Sättigungskapazität , Atomigkeit , Werthigkeit and eventually as Valenz . It is probably worth quoting Lothar Meyer at length from the first (1864) edition of Die Modernen Theorien der Chemie und Ihre Bedeutung für die Chemische Statik , where he wrote:
“The four groups of elements are usually distinguished according to a traditional, but not very well chosen, designation, as mono-, di-, tri- and tetraatomic or mono-, di-, tri- and tetrabasic . The former expressions are unsuitable because one cannot easily distinguish the usage from monatomic etc. atoms . The expressions monobasic etc. remind us that the doctrine of multiple saturation capacity derives its origin from Liebig’s classical investigations of polybasic organic acids. But since we are used to understand basic as the opposite of acid (in the chemical sense), this expression cannot be applied to the atoms either. However, it does not seem to be easy to replace it with another expression that is both comfortable and appropriate. The most correct and strictest way is probably to describe the atoms as those with 1, 2, 3 and 4 times the saturation capacity. The half-Greek and half-Latin expressions used by Wislicenus are shorter, namely “monaffin, di-, tri- and tetraffin”; in German one could also say “mono-, di-, tri- and quadrivalent”, for which, according to the judgement of tolerant philologists, “uni-, bi-, tri- and quadrivalent” could also be used. Characteristic and for many cases convenient is also the expression, according to which the four groups of atoms are usually described as having 1, 2, 3 and 4 “kinship units”. [ 95 ]

Edward Frankland (1825–1899) was the father of valence—or rather “combining power” as he phrased it. (Public domain image taken from https://en.wikipedia.org/wiki/Edward_Frankland ).
Note that in this translation, - werthig has been rendered as -valent and Verwandschaftseinheiten as associated units. By 1872, in the second edition of his book, Lothar Meyer was widely using the terms der chemische Werth and die Valenz [ 96 ]. This use of Valenz seems to be due to Carl Wichelhaus [ 97 ] who wrote “ Gebraucht man „Valenz” als kürzeres Wort an Stelle des von A. W. Hofmann eingeführten „Quantivalenz” in demselben Sinne, so ist es zunächst klar, dass zur Bestimmung der relativen Grösse dieser „atomfesselnden Kraft” nur diejenigen Verbindungen dienen können, welche ein Molecul repräsentiren ” (If one uses “valence” as a shorter word in place of the “quantivalence” introduced by A. W. Hofmann in the same sense, it is initially clear that only those compounds which represent a molecule can serve to determine the relative magnitude of this “atomic bounding force).
Another problem that confronted the early workers was that of variable valence. In 1852, Frankland recognized that some elements could have multiple valences [ 94 ]. For example, the group 15 elements formed compounds with combining power (valence) of three, such as NH 3 , PH 3 , and PCl 3 , but also with a combining power of five, as in NH 4 I and PH 4 I. Note that in these representations, no distinction is drawn between the number of atoms bonded to the group 15 atom (four) and the total number of additional atoms (five), as the ionic nature of these compounds was not explicitly recognized by Frankland. Frankland does not appear to have compared PCl 3 and PCl 5 , although both had been prepared earlier in the century [ 98 , 99 ].
August Kekulé ( Figure 7 ) proposed that elements had a fixed valence but, although he was aware of the work of Frankland, he did not recognize that combining power was the same as his valence [ 100 ]. This model of fixed valence was very successful in rationalizing the chemistry and the structures of organic compounds using a fixed valence of four for carbon, and allowed the extension to elements such as nitrogen and oxygen, which were proposed to have fixed valences of three and two, respectively. One of the successes of the model was that the fixed valence of four for carbon necessitated the presence of multiple bonds (or free valences). An early publication relating to chemical topology and graph theory was predicated upon the fixed-valence model for carbon, although the author, Oliver Lodge from University College London had, apparently, no great love for the word valency, and wrote, “Might I suggest the term “order” for non-chemical use, instead of atomicity or valency, which, though doubtless they do very well in chemistry, are not pleasant words? The change of zero might be made at the same time, and atoms of tetravalent atomicity be called atoms of the 2nd order, and so on” [ 101 ].

Friedrich August Kekulé (1829–1896) embraced the valence model and used it to rationalize organic chemistry (Public domain image taken from https://en.wikipedia.org/wiki/August_Kekulé/media/File:Frkekulé.jpg ).
Even Kekulé had to admit to reality and accept that some compounds did not fit well in his universal view and he introduced the description “molecular compounds” for substances that did not conform to his valence rules; “ A côté de ces combinaisons atomiques nous devons distinguer une seconde catégorie de combinaisons, que je désignerai par le nom combinaisons moléculaires” (In addition to these atomic combinations we must distinguish a second category of combinations, which I will refer to as molecular combinations) [ 102 ]. Taking PCl 5 as an example, Kekulé re-formulated the compound as PCl 3 , Cl 2 which evolved into the more modern dot notation PCl 3 ⋅Cl 2 .
By the latter part of the 19th century C.E., valence or one of its synonyms was sometimes being used in the sense oxidation number and sometimes to refer to the number of bonded atoms. The situation was well-summarized by Madan in 1869, “It is very much to be regretted that the subject of chemical nomenclature is in such an unsettled state. It seems a real reproach to chemists that scarcely two text-books can be found in which the same system of names is adopted, and that there is hardly a single number of a scientific periodical which does not contain specimens of totally different systems” [ 103 ].
3.2. Dalton and the Full Glory of Atomism
A crucial step in the development of our understanding of bonding is due to John Dalton (1766–1844). Instead of using alchemical symbols to describe real elements, philosophical elements and compounds [ 104 ], he proposed that elements should be denoted by symbols [ 105 , 106 ]. Furthermore, he recognized that these symbols could be combined to give representations of molecules and compounds that clearly identified the number and type of atoms of which they were composed. It is probably true to say that Dalton was responsible for the acceptance of the atomic theory by the broad body of scientists. His ideas were developed in 1803, but only broadly disseminated in his book A New System of Chemical Philosophy published in 1808. Dalton arranged his elements in order of atomic weight in a manner that is familiar to the modern chemist, although the atomic weights of the time differ from those we use now (H = 1, O = 7, and N = 5). The differences arise from Dalton’s assumption that, “When only one combination of two bodies can be obtained, it must be presumed to be a binary one, unless some cause appear to the contrary”. This assumption resulted in him formulating water and ammonia as HO and HN, respectively, with the consequence that the equivalent weights for combination with hydrogen were 1/2 and 1/3 of the “real” values. As mentioned earlier, Dalton used the term atom for both atoms and molecules (the atomic weight of ammonia, NH, was 6). The atomic symbols used by Dalton comprised a circle modified to uniquely describe an element. Although these were soon replaced by Jöns Jacob Berzelius with modern alphabetic symbols [ 107 , 108 , 109 , 110 , 111 ], Dalton’s contribution to bonding was to introduce a notation in which substances could be represented by combinations of atomic symbols. This implies a persistent interaction between the atoms—in other words, bonding. It is unclear whether Dalton considered that the representations were a precise representation of the spatial arrangement of atoms in molecules. Dalton clearly identified that spherical atoms of different elements could be different sizes but must have the same weight. In terms of shape, he is ambivalent and criticizes Berzelius’ view that “a compound atom cannot be considered as spherical, but that an elementary atom may be taken as such” [ 112 ]. On the one hand he states that he does not “see any reason sufficient for all simple atoms to be” spherical, and continues “those of hydrogen may be spherical perhaps; those of oxygen may be regular tetrahedrons; those of azote may be cylinders of equal diameter and altitude; &c, &c” [ 112 ]. Regarding compounds, his view is that, “Of all compound atoms, that consisting of 3 elementary atoms is probably most remote from a sphere, but when one compound contains 5 or more simple ones, the figure must, I should suppose, be virtually a sphere” [ 112 ]. Whether or not Dalton was convinced about the reality of the spatial arrangement, he clearly identified that the different arrangement of the atoms could define different compounds, using different arrangements of the atoms in the formula C 2 H 2 ON to describe albumen and gelatin ( Figure 8 ) [ 113 , 114 , 115 ].

( a ) John Dalton introduced atomic symbols as a part of his atomic model and ( b ) anticipated the occurrence of isomers in which atoms were arranged in different spatial manners (1829–1896) embraced the valence model and used it to rationalize organic chemistry [ 113 , 114 , 115 ]. (Image taken from https://www.biodiversitylibrary.org/bibliography/9535 ).
3.3. Isomerism … the Agony of Being the Same but Different
By the beginning of the 19th century C.E., chemists generally had an understanding that a single chemical formula represented a single compound, in part an unjustified extension of Proust’s law [ 116 ]. This was a view at odds with that expressed by Henry Cavendish before the nomenclature reforms of Lavoisier; Cavendish stated in 1787 that he thought it “very wrong to attempt to give [compounds] names expressive of their composition” [ 117 ]. This comfortable state of affairs was soon to end [ 118 ]. With the notation of Dalton and Berzelius in place, the constitution of compounds could be expressed by the combination of atomic symbols. In 1824 Liebig and Gay-Lussac described the preparation and properties of silver fulminate, Ag(CNO), which was a compound with interesting properties, “ Le fulminate d’argent ne détone jamais seul à la température de 100°, ni à celle de 130°; mais il faut éviter de l’exposer au plus léger choc entre deux corps durs, même lorsqu’il est dans l’eau ” (Silver fulminate never detonates alone at the temperature of 100° or 130°, but it should not be exposed to the slightest shock between two hard bodies, even when it is in water) [ 119 ]. In the same year, Friedrich Wöhler described silver cyanate ( cyansaure Silberoxyd ) and reported the analysis Ag(OCN)—in other words, the identical formulation to Liebig’s silver fulminate [ 120 ]. After a few exchanges between Liebig [ 121 ] and Wöhler [ 122 ], the former eventually conceded that the two compounds had an identical formulation but different properties [ 123 ]. Not content with upsetting the inorganic community, in 1828 Friedrich Wöhler proceded to cast confusion and shock into the organic and philosophical communities [ 124 ]. In purely chemical terms, Wöhler showed that syntheses designed to yield ammonium cyanate, [NH 4 ](OCN), gave the isomeric organic compound, urea OC(NH 2 ) 2 [ 125 , 126 , 127 , 128 ]. The philosophical challenge related to the doctrine of vitalism, which maintained that organic compounds possessed special properties because they contained a vital force as a result of being formed by living things [ 129 , 130 , 131 ]. Although the Wöhler urea synthesis was certainly one of the experiments that resulted in the questioning of the vitalism theory, it is now clear that it was by no means responsible for its discrediting, although this revision has not yet made its way to the chemical text-books [ 132 , 133 , 134 ]. The difference between silver fulminate and silver cyanate, with the modern formulations Ag(CNO) and Ag(OCN), respectively, leads irrevocably to the need for chemical bonding and the consequence that atoms have fixed interactions with specific other atoms and ultimately that the bonding of atoms implies a three-dimensional arrangement in space.
But we get a little bit ahead of the story. It took Berzelius to make the necessary leap of imagination. Berzelius had an array of disparate results available to him, which his genius correlated into one of the most important insights in the development of modern chemistry. Amongst these results were the cyanate/fulminate and ammonium cyanate/urea conundrums and in 1830, in a paper entitled Ueber die Zusammensetzung der Weinsäure und Traubensäure (John’s Säure aus den Vogesen), über das Atomengewicht des Bleioxyds, nebst allgemeinen Bemerkungen über solche Körper, die gleiche Zusammensetzung, aber ungleiche Eigenschaften besitzen , Berzelius proposed that these pairs of compounds should be described as homosynthetic or isomeric (Greek, ἰσομερής , equal part) bodies and favored the latter term [ 135 ].
3.4. Organic Chemistry and Structure—The Bond Reigns Supreme
After the insights of Berzelius, the stage was set for the growth of organic chemistry into a rigid intellectual discipline [ 136 ]. This is, unfortunately, not the place for a detailed discussion of the fascinating history of the representation of bonds in the development of organic chemistry [ 137 , 138 , 139 , 140 , 141 ].
The honor of introducing the first new type of bond probably goes to Alexander Butlerov (1826–1888) when he incorporated the double bond into organic structures. In doing this, he was also one of the pioneers of representing organic molecules with a two-dimensional structure connected by lines, representing the bonds or valences [ 142 ]. As Butlerov wrote, “Starting from the assumption that each chemical atom possesses only a definite and limited amount of chemical force (affinity) with which it takes part in forming a compound, I might call this chemical arrangement, or the type and manner of the mutual binding of the atoms in a compound substance, by the name of chemical structure” [ 143 , 144 , 145 ]. This can be seen as the beginning of the structural theory of organic chemistry [ 146 ]. An excellent overview of the competing claims and counter-claims for the discovery of structural formulae, the incorporation of multiple bonds and the genesis of the tetrahedral carbon atom has been recently published by Rocke [ 147 ].
Amid the claims and counter-claims, it is worthwhile seeing how bonds were interpreted in the general organic chemistry community at this time. Although the bonding had a topological meaning in terms of how the atoms were connected as the structural theory developed, the general understanding of chemists was that the depictions which correctly showed the connectivity, did not imply anything regarding the positions of the atoms in space [ 139 , 148 ]. For us, it is convenient to start the story with Archibald Scott Couper (1831–1892) [ 149 , 150 , 151 ] and August Kekulé [ 100 ] who almost simultaneously proposed that tetravalent carbon atoms could link together to form chains with C–C bonds, building on Charles Gerhardt’s ideas about homologous compounds differing by the addition of CH 2 moieties [ 152 ]–and so was modern organic chemistry born! Alexander Crum Brown (1838–1922) had introduced his croquet-ball notation (which persists to this day with the convention of white, red, black, and blue colorations for hydrogen, oxygen, carbon, and nitrogen atoms, respectively) for representing chemical structures in 1864 [ 31 , 153 , 154 ]. In these structures, the four lines to a carbon atom represent the valency of four and do not imply any specific spatial structure. Crum Brown and Frankland were at pains to emphasize this point. Hein stated, Kekulé’s theories “required no specific arrangement in space but did refer to chemical relations between atoms in three dimensions [ 155 ]. However, as the 1860s developed, Kekulé was shifting his ideas more and more to atoms having a distinct spatial as well as valence relationship. In the models he used to represent molecules, a saturated carbon atom was represented by a tetrahedron. By 1865, Kekulé had proposed the hexagonal structure for benzene and had also identified different isomers of the disubstituted derivatives [ 156 , 157 ].
Although Kekulé had perhaps opened the floor for the discussion of the three-dimensional arrangement of molecules, it was left to Jacobus Henricus van’t Hoff (1852–1911) [ 158 , 159 , 160 ] and Joseph Achille Le Bel (1847–1930) [ 161 ] to independently demonstrate that a three-dimensional, tetrahedral carbon atom was needed to explain aspects of stereochemistry related to stereogenic centers and chiral molecules. In particular, van’t Hoff used his ideas to explain the stereochemical consequences of cumulated double bonds in allenes and cumulenes [ 162 ]. Although the new ideas were not immediately accepted, their popularization and championing by Johannes Wislicenus (1835–1902) resulted in their eventual acceptance [ 163 , 164 ].
From the middle of the 19th century C.E., bonds were routinely being represented with the lines that we are familiar with today, although it is also fair to say that there was little understanding or consensus as to what the lines actually meant. The prevalence of the Kekulé view and its emphasis on a fixed valence of four for carbon was instrumental in this development. One debate, which is again beyond the scope of this article, was related to the question of whether the depiction of the bonds implied anything about the spatial arrangement of the atoms. Slowly the organic chemistry community came to accept this, with the critical observations relating to isomerization and the need for a fixed three-dimensional structure. An excellent account is given in Ramberg’s book Chemical Structure, Spatial Arrangement. The Early History of Stereochemistry, 1874–1914 [ 90 ].
3.5. Strings and Things—Jørgensen and Coordination Chemistry
The next part of our story begins in 1839, with Charles Gerhardt (1816–1856) introducing his model of organic chemistry, which consisted of the making and breaking of bonds between residues. In reality, Gerhardt’s residues were closely related to radicals. He originally called the process of joining the residues together copulation, but later used the description “double decomposition” [ 165 , 166 , 167 , 168 , 169 , 170 , 171 , 172 ]. Although Gerhardt originally used this for describing salts of organic acids, the model was then further extended, in particular by Jakob Berzelius to cover a more general linkage in a pairwise manner or into chains of copulated compounds ( Paarlinge oder gepaarte Verbindungen ) [ 173 ]. This developed most successfully in the organic arena to the chains of carbon at the core of the structural theory that we discussed in the previous sections.
However, we now see another of the deviations from narrative linearity that litters the course of chemical history with the attempts to extend the chain model to inorganic chemistry, in particular to coordination compounds. Berzelius used his annual reviews of progress in science to extend the copulation model and its C–C bonds which rationalized organic chemistry to N–N bonds in metal complexes, denoting [Ni(NH 3 ) 6 ]Cl 2 as NiCl + 3NH 3 in which the strike-through means that the ammonia is copulated in a chain (note the atomic and equivalent weights used by Berzelius gave the formula quoted and also Berzelius’ use of raised integers to denote composition [ 174 ]. This approach was initially embraced by the inorganic community as it provided some structure to the study of the poorly understood coordination compounds. It fell to Blomstrand to further develop and expand the Berzelius ideas and in Die chemie der Jetztzeit vom standpunkte der electrochemischen Auffassung. In Aus Berzellius Lehre entwickelt, he introduced a new notation for the copulated ammonia molecules and extended the system to other ligands such as cyanide in K 4 [Fe(CN) 6 ] [ 175 ]. Although an interesting historical aside, and not so fanciful as they initially appear to our modern eyes, these structural representations evolved in the absence of any knowledge of what a bond might actually be and before the electron had been discovered. Nevertheless, the model had the major deficit that it was unable to predict how many of a particular ligand might be associated with a particular metal salt.
The Blomstrand model was adopted by Sophus Mads Jorgensen and used to rationalize a vast body of experimental work and precise observations reasonably well [ 176 , 177 , 178 ]. Jorgensen accounted for the valence in compounds such as [Co(NH 3 ) 6 ]Cl 3 by proposing three separated chains of ammonia ligands each terminated with a chlorine [ 179 ]. There remained three difficulties which were not addressed: (i) the nature of the interactions between the ligands and or atoms in the conjugated chain were unknown, (ii) there was no rationale what molecules could act as ligands, and (iii) at the time that organic chemistry was developing an awareness of the third dimension, this was neglected in the coordination compounds.
3.6. Primary and Secondary Valences—Or When is a Bond not a Bond?
The Jörgensen ideas were eventually replaced by Werner’s coordination model. It is not appropriate to rehearse the controversy between these two scientists in this article [ 180 , 181 , 182 ], but we should note that Werner was influenced by the developing three-dimensional understanding of organic chemistry and developed a new way of rationalizing the structure of coordination compounds. In 1893, Alfred Werner proposed his model in Beitrag zur Konstitution anorganische Verbindungen [ 183 ]. He surveyed the literature of coordination compounds, in particular the work of Jørgensen, and rationalized the compounds in terms of a primary valence ( Hauptvalenz ) and a secondary valence ( Nebenvalenz ). These are equivalent to oxidation state and coordination number, respectively. This topic has been discussed in detail elsewhere [ 184 ]. Now that the detour into chain theory of inorganic compounds has been corrected, we can return to our main theme and the birth of the modern understanding of bonds and bonding.
4. The Modern Era
4.1. the advent of the electron and ions.
Electricity has a long and noble history, with empirical observations dating back to the ancient civilizations of Egypt and Greece [ 185 , 186 , 187 , 188 , 189 , 190 , 191 ]. This is not the place to relive these early experiences, ranging from electric shocks from fish and reports on the phenomenon of static electricity, nor is it appropriate to enter into the debate regarding the function of what is claimed to be a galvanic cell dating to Parthian era (250 B.C.E.–230 C.E.) [ 192 , 193 , 194 ]. For us, the story of the electron properly starts in 1600, when William Gilbert [ 195 , 196 , 197 ] published his work on magnets and magnetism [ 198 ]. In this book, Gilbert serendipitously connects magnetism and electricity as he distinguishes between the magnetic effect, typified by the behavior of lodestone, and static electricity as exhibited by substances such as amber. To Gilbert, we owe all the modern electr - words, including electric, electricity and electron; Gilbert derived the word electricus from the Latin electrum and Greek ἤλεκτρον (elektron) meaning amber and used it adjectivally in de Magnete , “ Vim illam electricam nobis placet appellare ”. (We prefer to call it electric power). As early as 1620, Francis Bacon devoted a section of his Instauration Magna Part III: The Phenomena of the Universe; or a Natural and Experimental History for the foundation of Philosophy, to electrical phenomena (Electricity: 1. The bodies that are electrical; 2. The bodies that are not electrical; 3. The bodies disposed to be attracted; 4. Leading experiments made with electrical bodies) [ 199 ].
The recognition that the electrical phenomenon was associated with two different types of effect emerged in the course of the 18th and 19th centuries C.E. [ 200 , 201 , 202 ], commencing with the observation by Charles François de Cisternay du Fay (1698–1739) that silk and amber had opposite attraction or repulsion properties, and interpreted this is in terms of their possessing different fluids [ 203 , 204 ]. The transition from a fluidic vision of electricity to one involving charges was made by Benjamin Franklin in 1747, when he introduced the terms positive and negative [ 205 ]. These terms still related to a fluidic model and referred to and excess or a deficit of an electrical fluid. Thus, began a debate about the direction of flow of electrical charge that mystifies students to this day. In the United States, Ebenezer Kinnersley [ 206 ] made similar observations to du Fay, describing them in a 1762 letter to Benjamin Franklin in which he uses the latter’s negative and positive notation [ 207 ].
The first association of the electrical fluid with atomic and molecular structure seems to stem from the work of Richard Laming [ 208 , 209 , 210 , 211 ]. He developed a model composed of central atoms surrounded by electrical particles in which “different sorts of atoms are naturally associated with unequal quantities of electricity”—rephrase this as “atoms of different elements are associated with different numbers of electrons” and you have a very modern view of atomic structure [ 211 ]. Laming considered atoms to be solid, a view that was contested by Sloggett, who otherwise had a very similar vision of atomic structure [ 212 ]. As the 19th century C.E. progressed, the concept of a charged particle that behaved like an atom of electricity became established [ 200 , 213 ], and it fell to George Johnstone Stoney to propose the name electrine for this particle in 1881 [ 214 ]. Stoney was also one of the first (if not the first) to recognize that there was a fundamental unit of electricity and his work seems to have predated that of Hermann Ludwig Ferdinand von Helmholtz [ 215 ]. Although first published in 1881 [ 214 , 216 ], the text in which Stoney proposes a fundamental unit of electricity is that of a lecture presented in 1874 [ 217 ].
We now come to the electron [ 202 ]. The name electrine, proposed in 1881 [ 214 , 216 ] was widely ignored by the community and Stoney modified this to electron in 1894 [ 213 ]. The Helmholtz memorial lecture given by George Francis Fitzgerald is remarkable for a number of reasons [ 218 ]. Fitzgerald talks extensively about electrons, but nevertheless writes, “The suggestion that electrons have an individual existence is undoubtedly tempting, but it is worth while keeping constantly in view the possibility that their constancy of quantity is connected with a constancy of structure of the ether rather than with any individual existence of each electron” [ 218 ]. Like Helmholtz, he relates the electron to chemical bonding, but once again, there is a conflict with modern electrostatic ideas when he writes, “This follows from considering that the work done in the combination of H and Cl may be mostly due to the attraction of electrons” [ 218 ].
One year after Fitzgerald cast doubts about the individual existence of the electron, Joseph John Thomson ( Figure 9 ) reported their experimental observation [ 219 ]! Thomson was studying the emanations of the cathode ray tube, originally described by William Crookes, and performed experiments indicating that cathode rays really were unique particles. At the time, a debate was raging as to whether the rays were waves, atoms, or molecules, but Thomson made the case for cathode ray particles and provided estimates of their charge and mass, the latter being very much smaller than that of the lightest known atom, hydrogen. Thomson described the phenomenon and the new corpuscles thus: “If, in the very intense electric field in the neighbourhood of the cathode, the molecules of the gas are dissociated and are split up, not into the ordinary chemical atoms, but into these primordial atoms, which we shall for brevity call corpuscles; and if these corpuscles are charged with electricity and projected from the cathode by the electric field, they would behave exactly like the cathode rays” [ 219 ]. An excellent introduction to the scientific background and the prevalent scientific thought prior to his discovery is presented in Thomson’s Nobel Prize address from 1906 [ 220 ]. By 1908, the electron was sufficiently established as a reality in chemistry, that William Ramsay could give a lecture entitled The Electron as an Element as his presidential address to the Royal Society of Chemistry [ 221 ].

Sir Joseph John Thomson (1856–1940), the discoverer of the electron. Once the electron had been discovered, the time was ripe for modern theories of bonding to emerge. (Public domain image taken from https://en.wikipedia.org/wiki/J._J._Thomson#/media/File:J.J_Thomson.jpg ).
The discovery of the electron and its importance in the understanding of chemical bonding cannot be over-emphasized. The history of chemical bonding can with justification divided into a pre-history before the electron and the modern era post-1897.
4.2. Ionic Bonding
Now that the electron has entered our vocabulary, we can move directly to a discussion of bonding in modern terms. We will jump over the discovery of the proton and the neutron, together with the development of the atomic structure model with a small central nucleus surrounded by electrons [ 222 , 223 , 224 , 225 , 226 ]. These are critical developments in the history of modern chemistry, but only tangential to our main theme of bonding, which is to do with electrons rather than nuclei. Indeed, it is fair to say that most historical studies of atomic structure completely ignore the issue of bonding.
Ionic bonding fits perfectly within the IUPAC definition of bonding [ 1 ], although it might cause conceptual difficulties for those embedded within the covalent world of shared electrons. The ionic bond is defined by the electrostatic interaction between negatively and positively charged chemical species. The latter are, in turn, defined by the transfer of an electron or electrons from one atom to another. It is worth making a comment here that “pure” ionic bonding is an ideal concept: in reality, there will always be a finite, but often vanishingly small, probability of finding the “transferred” electron or electrons close to their mother atom. In other words, there will always be a degree of covalency. We discuss this aspect later.
Ions had become familiar to chemists in the course of the 19th century C.E. Michael Faraday first demonstrated that solutions of certain compounds in water could conduct electricity. He proposed that the action of the electricity caused the compounds to break up into charged particles which were responsible for the conductivity. In his early experiments, he does appear to have considered that the ions might be present in the parent compound itself. Farady also introduced the new term ion (Greek, wanderer) and recognizing that the ions had charges, also introduced the descriptions cation and anion.
It was another half century before Svante August Arrhenius made the important proposal that the charge carrying species, the ions, could be atoms carrying a positive or negative charge. It was also Arrhenius who recognized that formation of the cations and anions in aqueous solution was a result of the dissolution itself and not, as Faraday had proposed, through the action of electricity.
The discovery of the electron by Thomson was critical to the understanding of ions and ionic bonding and Thomson went as far as suggesting that in a compound like HCl, the atoms were joined by an electromagnetic force, “There seems to me to be some evidence that the charges carried by the corpuscles in the atom are large compared with those carried by the ions of an electrolyte. In the molecule of HCl, for example, I picture the components of the hydrogen atoms as held together by a great number of tubes of electrostatic force; the components of the chlorine atom are similarly held together, while only one stray tube binds the hydrogen atom to the chlorine atom”; he also proposed that HCl had a permanent dipole, with positive and negative ends [ 219 ]. Thomson returned to this theme in his 1904 book Electricity and Matter , when he wrote, “If we interpret the ‘bond’ of the chemists as indicating a unit Faraday tube, connecting charged atoms in the molecule, the structural formulae of the chemist can be at once translated into the electrical theory. . . but the symbol indicating a bond on the chemical theory is not regarded as having direction, no difference is made on this theory between one end of the bond and the other. On the electrical theory, however, there is a difference between the ends, as one corresponds to a positive, the other to a negative charge” [ 227 ]. Interestingly, the word bond only occurs on a very few occasions in this book, but it is clear that he is now talking explicitly about bonding in ionic terms. In the example of ethane, Thomson envisages negatively-charged hydrogen and positively-charged carbon atoms linked by “Faraday tubes”. After starting the discussion of ethane with a model equivalent to [(C 2 ) 6+ (H − ) 6 ] where each carbon bears a 3+ charge, he then attempts to rationalize the C–C interaction in terms of Faraday tubes (electrostatic interactions) between these two atoms, resulting in a chemical inequivalency of the atoms, one bearing a 4+ charge and the other 2+. Thus, the framework was in place for an understanding of ionic bonding, or as it was described at the time “polar bonding”, in the first decade of the 20th century C.E. The model was proposed as a universal one for all compounds, even those that did not form ions in solution. Although some of Thomson’s proposals have more to do with covalent bonding, they serve to remind us that from the earliest period, chemists were aware of the dichotomy and inherent difficulties asociated with the distribution of electrons in bonds and in molecules. It took Linus Pauling another 30 years in the future, to introduce the concept of electronegativity.
Another beginning for the modern concept of ionic bonding lies with Gilbert N. Lewis ( Figure 10 a), whose earliest published work on bonding was in 1913 [ 228 ]. However, in 1923 Lewis stated that he made the first of his models based upon the distribution of up to eight electrons to the vertices of a cube in 1902. In papers of 1913 [ 228 ] and 1916 [ 229 ], Lewis gave a picture of the ionic bond similar in many respects to that of Thomson. Lewis recognized that a stable octet could result either from the sharing of electrons or by the transfer of electrons from one atom to another. He represented the arrangement of eight electrons (the octet) at the vertices of a cube. In his representation, atoms of sodium and chlorine have, respectively, one and seven electrons at these vertices. He postulates the stability of full or empty octets, which can be achieved by the transfer of one electron from the sodium atom (generating an empty octet) to the chlorine (generating a full octet) and building ionic sodium chloride.

The credit for the development of the modern conceptual models of bonding goes to ( a ) Gilbert Newton Lewis (1875–1946) who placed electrons to be shared at the vertices of a cube (thus conforming to the octet model) and ( b ) Walther Ludwig Julius Kossel (1888–1956) who considered the complete transfer of electrons in ionic bonds. (Images taken from https://en.wikipedia.org/wiki/Gilbert_N._Lewis#/media/File:Lewis-cubic-notes.jpg and https://en.wikipedia.org/wiki/Walther_Kossel#/media/File:Kossel,Walther_1928.jpg ).
As Lewis was developing his ideas in the United States and Thomson was working in the United Kingdom, Walther Kossel ( Figure 10 b) was following a similar direction in Germany. In 1916, he also identified the special stability of the closed-shell configuration of the elements of group 18 and drew attention to the more general relationships of these closed-shell configurations to those of stable ions [ 230 ]. In this paper, Kossel also identifies the positive and negative valencies that can be associated with a particular atom, for example carbon with four valence electrons could lose four electrons to give C 4+ or gain four electrons to generate C 4− , both of which are closed-shell configurations “ Jedes Element besitzt sowohl eine positive wie eine negative Maximalvalenz, die sich stets zur Zahl 8 summieren, und zwar entspricht die erstere der Gruppennummer ” (Each element has both a positive and a negative maximum valence, which always add up to the number 8, the former corresponding to the group number) [ 230 ]. In this remarkable paper, Kossel, like Thomson and Lewis also identifies the importance of the octet in compounds that we would today describes as covalent (H 2 O, CO 2 , NH 3 , HBr, and CH 4 for example). These themes were further developed and expanded by Kossel from 1919 onwards. In 1919, Kossel recognized that the formation of stable ions was not limited to the attainment of an octet, but in the heavier elements an 18-electron configuration had a similar stability, typified by the sulfide anion S 2– . In subsequent papers, also published in 1919, Kossel expanded his vision to both covalent and ionic bonds, discussing carbon compounds and stating, “The behavior of carbon in exhibiting a constant valency, previously considered as particularly simple and typical, must be regarded as exceptional when compared with the majority of elements in which the polar character is marked. In general, a distinction must be made between heteropolar and homopolar linkings” [ 231 , 232 ]. Kossel also extended his model to include coordination compounds and addressed the variable valence exhibited in compounds such as PCl 3 and PCl 5 .
Although the discovery of ionic bonding is usually attributed to Lewis and Kossel, Thomson should be regarded as an important contributor to the ideas and concepts. However, both Thomson and Kossel acknowledge a debt to Richard Abegg who arguably pointed all three in the direction of the octet. In a 1904 paper entitled Die Valenz und das periodische System. Versuch einer Theorie der Molekularverbindungen (Valence and the periodic system. An attempt at a theory of molecular compounds) [ 233 ], Abegg noted that for a given element, the sum of the absolute value of its maximum negative valence (such as −2 for sulfur in H 2 S and its maximum positive valence (such as +6 for sulfur in H 2 SO 4 ) is usually equal to 8. Abegg was using “valence” in the sense of oxidation number and concludes, “The sum of eight for our normal and contravalences then receives the simple meaning of a number that represents the points of attack of the electrons for all atoms, and the group number or positive valence indicates how many of the eight points of attack electrons must hold in order for the substance to appear as an electron neutral element, the ‘positive’ elements need few (1–3), the ‘negative’ many (5–8) electrons” [ 233 ]. Abegg returned to the theme on a number of occasions, developing models that paralleled those being expounded by Thomson, Kossel, and Lewis [ 234 , 235 ]. In 1905 he expanded his ideas to a more general valence theory, and most importantly, clearly identified the confusion arising by the parallel usage of valence and affinity “as soon as the sharp distinction between valence and affinity, which seems necessary to us, is made …almost all contradictions are transformed into agreement” [ 234 ]. Also in 1905, Abegg addressed the question of higher oxidation, and stated, “Higher levels of connection can only occur here because these elements do not actuate their negative (“normal”) valences, but their numerous positive (“contra”) valences and thus function eo ipso basic or, more precisely, positive” [ 235 ].
4.3. Covalent Bonding—The Legacy of G.N. Lewis
The relationship between the number of electrons in an atom and the types of compounds it forms in combination with other atoms together with its valence was clearly identified by Thomson [ 236 ] and others. As early as 1914, Thomson had realized that there were compounds for which his electrostatic ionic model was not appropriate and considered an alternative in which electrons held the atoms together through a sharing, “When the atoms are electrically neutral, i.e., have no excess of positive over negative charge or vice-versâ , for each tube of force which passes out of an atom, another must come in; and thus each atom containing n corpuscles [electrons] must be the origin of n tubes going to other atoms and the termination of n tubes coming from other atoms” [ 237 ]. This is essentially the description of a covalent bond with one important exception—for Thomson, a bond seems to be associated with a single electron and a double bond results when two tubes are formed. In a 1921 paper, Thomson clearly identifies the importance of the octet and explicitly relates the arrangement of the eight electrons in an atom to a regular octagon; furthermore, in a manner resembling Lewis, he describes the formation of compounds thus: “Whereas all the plane faces of a cube are four-sided, the twisted polyhedron has 8 triangular faces as well as 2 four-sided ones, thus two such polyhedra could be placed so as to have either 2, 3, or 4 corners in common” [ 236 ]. In this same paper, he addresses the question of variable valency in compounds such as PCl 3 and PCl 5 . Thomson’s views on bonding and other chemical matters are best summarized in a series of lectures from 1923 entitled The Electron in Chemistry in which, once again chemical bonding is represented by the fusion of polygons through vertices and edges and faces [ 238 , 239 , 240 , 241 ].
Covalent bonding, defined in terms of two-electron two-center bonds between atoms, is usually thought to originate in the 1916 publication by Gilbert N. Lewis [ 229 ], although the roots are to be found in the earlier 1913 paper [ 228 ]. In his 1916 paper, Lewis visualizes the octet by locating electrons at the vertices of a cube centered on the atom and then builds molecules (or rather bonds) through the sharing of vertices, edges or faces between cubes centered on the bonded atoms. He also introduces the classical Lewis dot structures in this same publication. Although the concept was first formulated by Lewis, it did not excite much attention in the chemical community. The real credit for the general acceptance and adoption of the covalent model goes to Irving Langmuir [ 242 , 243 , 244 , 245 , 246 , 247 ] and in particular his 1919 paper entitled The Arrangement of Electrons in Atoms and Molecules . In this 66-page manuscript, Langmuir retells the story of the Lewis description in terms of a number of postulates and also clearly identifies the change from an octet to a stable 18-electron configuration that can occur in the heavier elements. Equally important was the paper Polarity and Ionization from the Standpoint of the Lewis Theory of Valence by Wendell Latimer and Worth Rodenbush in which they relate polarity and reactivity to the bond types exemplified in the Lewis model [ 248 ]. In his 1923 monograph, Lewis benefits from the recasting by Langmuir and presents a masterful summary of his theory and also establishes that his original ideas, first published in 1913 and 1916, dated as far back as 1902 [ 249 ]. Perhaps one of the best overviews of the state-of-the-art in the understanding of bonding in 1923 is found in the various publications arising from a meeting of the Faraday Society of the Royal Society of Chemistry in that year [ 250 , 251 , 252 , 253 , 254 , 255 , 256 , 257 , 258 , 259 ].
Lewis does not use the words covalent or covalency in his 1916 publication [ 229 ]. By the beginning of the 1920s, ionic and covalent compounds were being generically described as having polar or non-polar links, the latter referring to the sharing of two electrons between linked atoms [ 260 ]. Nevertheless, by 1923, Fowler [ 257 ], Lowry [ 255 , 258 ], and Sidgwick [ 260 ] were all using these words in more-or-less the modern sense, apparently with the assumption that the reader understood them and their context. Where did they come from? Here we have, once again, to thank Irving Langmuir [ 242 , 243 , 244 , 245 , 246 , 247 ], for not only did he popularize the Lewis octet model and mediate its general acceptance in the chemical community, but he also introduced a nomenclature appropriate to the model. In his first 1919 paper he writes: “It is therefore proposed to define valence as the number of pairs of electrons which a given atom shares with others. In view of the fact known that valence is very often used to express something quite different, it is recommended that the word covalence be used to denote valence defined as above” [ 242 ]. As an aside, we note that Lewis introduced the word photon in 1926 [ 261 ].
As a short summary of these sections, it is worth emphasizing that the absolutism of the early part of the 20th century C.E. in dividing bonds into ionic or covalent has now been significantly moderated and a continuum between almost purely ionic and entirely covalent (in diatomic X 2 species) is generally recognized.
4.4. It’s All to do with Quantum
This article is not a history of quantum mechanics, but rather one of bonding. The fundamental development of quantum mechanics is dealt with in other excellent works [ 262 , 263 , 264 , 265 , 266 , 267 , 268 ] and we are only concerned with the transition from atoms to molecules. Nevertheless, it is not possible to underestimate the impact that the “new” quantum mechanics had on chemistry at the beginning of the 20th century C.E. It all started with Erwin Schrödinger who, after initial work on the consequences of the Bohr atom [ 269 ], published the basis of his eponymous equation in 1926.
Although exact analytical solutions could be obtained for the hydrogen atom, for larger systems with three or more particles (two or more nuclei or one nucleus and two or more electrons), it was not possible to calculate exact solutions for the Schrödinger equation. Nevertheless, the precision of the exact solutions for the hydrogen atom stimulated research into approximations for the multi-body problem.
The first published study of a polyatomic species using quantum mechanics was on dihydrogen and published by Walter Heitler and Fritz London in 1927 [ 270 ]. Heitler subsequently developed the approach to the study of homoatomic bonds and addressed the questions of ground state and valence state electron configurations [ 271 , 272 , 273 , 274 ]. The introduction of approximate analytic atomic wave functions for atoms other than hydrogen by researchers including John C. Slater [ 275 ], Carl Eckart [ 276 ], Linus Pauling [ 277 ], and Clarence Zener [ 278 ] was a critical advance in extending the quantum mechanical description of bonding to molecules of chemical relevance. As we see in the following section, this approach uses hydrogen-like atomic orbitals to generate localized bonds.
At the same time as the Heitler–London approach was proving scientifically and intuitively successful, an alternative quantum mechanical approach, molecular orbital theory, was being developed [ 279 , 280 , 281 ]. This approach was pioneered by Robert S. Mulliken [ 282 , 283 , 284 , 285 , 286 , 287 , 288 , 289 ], John Lennard-Jones [ 290 , 291 , 292 ], and Friedrich Hund [ 293 , 294 ] and ultimately described the bonding not in terms of localized bonds between two atoms but multi-center bonds over many atoms. The first calculations using this new method were on H 2 + and H 2 .
By the end of the 1920s, two approaches were dominating—the multi-center molecular orbital approach and the localized-bonding model using hydrogen-like atomic orbitals (what we now describe as the LCAO, linear combination of atomic orbitals model). Despite the applicability of the results to chemistry, the majority of the active workers in this field came from physics. The results were regarded with great interest by the chemical community, but were probably not embraced as methods and techniques that would impinge on the practice of the working synthetic chemist. This was soon to change!
4.5. It’s More than just s and p—The Pauling Era and the Triumph of Valence Bonds
By the second decade of the 20th century C.E., Niels Bohr and Ernest Rutherford had developed a model for the atom comprising electrons orbiting a small central nucleus [ 295 , 296 , 297 , 298 , 299 , 300 , 301 ]. The Bohr model was predicated upon a number of assumptions: (i) electron(s) move in stable orbits around the nucleus, (ii) these stationary orbits are maintained at fixed distances from the nucleus, and (iii) the electron(s) can occupy no orbit other than these. Bohr calculated the energies of these orbits for the hydrogen atom. This allowed Bohr to correlate his calculated energies (and more critically the energy differences between his orbits) with the results obtained by spectroscopists studying the atomic spectrum of hydrogen. Rydberg [ 302 , 303 ], Balmer [ 304 ], and Ritz [ 305 ] had convincingly shown that the wavelengths of the lines in the atomic spectrum of hydrogen could be calculated by a simple equation involving a constant (the Rydberg constant) and two integers n 1 and n 2 , which Bohr equated with his orbits [ 306 ]. Subsequent refinements resulted in the identification of sub-shells within each orbit with n > 1. Spectroscopists had introduced the descriptors sharp, principal, diffuse, and fundamental to describe lines in atomic spectra, and Hund used these, with the abbreviations s , p , d , f , to define these new subshells [ 307 ]. This is the origin of the familiar modern description of s , p , d , and f (etc.) applied to atomic orbitals [ 308 , 309 ].
The use of hydrogen-like atomic orbitals created a problem for the “real” chemist, who “knew” that the four C–H bonds in methane were identical. The molecular orbital method constructed different types of multicenter orbitals involving either the 2 s or the 2 p orbitals interacting with the hydrogen 1 s orbitals.
The approach developed by Heitler and London evolved into valence bond theory, often described as the Heitler–London–Slater–Pauling model [ 310 , 311 ]. Linus Pauling ( Figure 11 ) published his first paper on bonding in 1928 in which he correlated the Lewis model with quantum chemical calculations [ 312 ]. In 1931, he emerged as the champion of valence bond theory and explained a multitude of chemical features in theoretical terms that were also accessible and relevant to the broader chemical community. His subsequent publication The Nature of the Chemical Bond. Application of Results Obtained from the Quantum Mechanics and from a Theory of Paramagnetic Susceptibility to the Structure of Molecules extended the quantum mechanical treatment of the electron-pair bond to elements heavier than hydrogen [ 313 ]. Pauling also used the quantum mechanical approach to describe strong hydrogen bonds and most importantly, changing the electronic configuration of ground-state atoms to allow the generation of valence bond orbitals. For example, he postulates the change from a 2 s 2 2 p 2 configuration for carbon in the ground state to 2 s 2 p 3 in the valence state, which is then used to construct a set of four equivalent tetrahedrally disposed eigenfunctions and uses these to explain the four equal covalent bonds in methane. He also used the valence bond approach to return the Werner model for coordination compounds. How could a transition metal bind six ligands? He started with the set of five d orbitals and observed that three of these ( d xy , d xz , d yz ) are not optimized for bond formation in octahedral complexes and subsequently constructed a set of six hybrid orbitals from the s , p x , p y , p z , d x2–y2 and d z2 orbitals oriented towards the vertices of an octahedron. This resulted in a consistent valence bond description of transition metal complexes. The conclusion of this paper is insightful and clearly indicates his early appreciation of the continuum between ionic and covalent bonding by stating that “the transition-group elements almost without exception form electron-pair bonds with CN − , ionic bonds with F − , and ion-dipole bonds with H 2 O; with other groups the bond type varies”. He subsequently developed idea of the unequal distribution of electrons within a chemical bond and introduced the concept of electronegativity [ 314 ]. The terms hybrid orbital and hybridization were introduced by Van Vleck in 1933 [ 315 ]. Nevertheless, the hybrid model involving metal s, p , and d orbitals was not without its difficulties. In particular, Pauling had to select between “inner” (3 d ) and “outer” (4 d ) orbitals to reproduce the electronic properties of high and low spin first row transition metal complexes.

Linus Carl Pauling (1901–1994) probably did more to transform the understanding of bonding than any other scientist and the legacy of his paradigm-shift book The Nature of the Chemical Bond lives on today. (Public domain images taken from https://en.wikipedia.org/wiki/Linus_Pauling#/media/File:Linus_Pauling_1948.png ).
Pauling’s vision of chemistry is best presented in the three editions of The Nature of the Chemical Bond , which provide not only his understanding and vision of chemical bonding, but also show how it evolved with time [ 316 , 317 , 318 ]. The hybridization model that he developed for transition metal complexes rationalized the bonding in octahedral and square-planar complexes and provided a link to crystal field theory.
The crystal field theory originated in a publication of Hans Bethe from 1929 [ 319 ] in which he investigated the influence of an electric field on an atom. He demonstrated that the terms of the atom split dependent on the symmetry of the field and the angular momentum of the atom. This was the basis of the crystal field theory subsequently developed to describe the behavior of electrons in d -orbitals. The model is purely electrostatic and ligands are treated as negative point charges that have a repulsive interaction with electrons in the metal atom or ion. The Bethe paper limited itself to effects in crystals and did not extend to the general case of coordination compounds. In 1932, Van Vleck extended the concept to a new crystal field theory [ 320 ] applied specifically to transition metal compounds which, on the one hand, proved to be incredibly successful but on the other hand was predicated on a physical model that completely ignored chemical bonding.
4.6. The Triumph of the Molecular Orbital Model in Organic Chemistry
At the same time as Pauling was developing his valence bond ideas, Erich Hückel (1896–1980) [ 321 ] was applying molecular orbital theory to unsaturated hydrocarbons [ 322 , 323 , 324 , 325 ]. In this work, he concentrated upon the π-bonding rather than σ-bonding in molecules and developed a model that was embraced by the organic chemistry community for its elegance, (relative) conceptual and computational simplicity, and ability to explain the properties of important unsaturated compounds such as the aromatic hydrocarbons [ 326 , 327 , 328 ]. We owe the ubiquitous and iconic picture of the doughnut-shaped electron clouds above and below the planar benzene molecule to Hückel and the success of his model. Mathematically, the Hückel approach is an LCAO method which is close to a free-electron model with the electrons moving in a one-dimensional box. Being mathematically rather simple, the basic Hückel method allowed non-computational chemists to make qualitatively accurate and chemically useful descriptions of simple unsaturated molecules. As the approach became more sophisticated, it became a powerful tool in developing theories of aromaticity and anti-aromaticity [ 329 , 330 , 331 ].
Although the great success of Hückel theory is associated with organic chemistry, the model has been extended to inorganic systems and the solid state, notably by Roald Hoffmann [ 332 ].
5. Quantification
5.1. when calculation moved from pen and paper to machines.
Early quantum mechanical calculations were performed by hand or using simple mechanical devices. The development of the electronic computer after the second world war had an enormous influence on computational chemistry. In the 1930s, Douglas Hartree was developing the use of mechanical differential analyzers, which could be applied in the numerical solution of Hartree-Fock calculations [ 333 ]. In the post-war period, one of the first electronic computers to be used for molecular quantum chemistry calculations was the Manchester Mark II, with the earliest publications appearing in the mid-1950s [ 334 , 335 , 336 , 337 ]. This was one of the multiple beginnings of computational chemistry in the United Kingdom. In parallel, the influence of Lennard-Jones in Cambridge ensured that the interest in computational chemistry was high. After the war, Hartree and Maurice Wilkes were ensconced in Cambridge and a thriving computational chemistry group developed, with the first publications also appearing in the mid-1950s [ 338 , 339 , 340 , 341 , 342 , 343 , 344 , 345 , 346 ]. Boys had identified the need for computational methods in a 1950 paper where he wrote with considerable perspicacity: “It is shown that the only obstacle to the evaluation of wave functions of any required degree of accuracy is the labour of computation” [ 347 ].
The United States was equally active and an overview of molecular orbital theory published by Roothaan in 1951 explained the approach and the mathematical requirements so clearly, that it was inevitable that a computational chemistry effort would develop [ 348 , 349 , 350 ]. The Roothaan paper over-shadowed a very similar manuscript published by Hall in the United Kingdom [ 351 ]. Nevertheless, the development in the United States seems to have been a little slower than in the United Kingdom [ 352 ]. Parr and Crawford in their report on the 1951 meeting Quantum-Mechanical Methods in Valence Theory note that, “Slater cautioned that direct attack on problems of electronic structure is not yet within the reach of automatic computing machines; the machines can do much complex arithmetic, but the problem of the molecule has not yet been reduced to an arithmetic level”, although they also give progress reports on work in Cambridge, Chicago, and other centers [ 353 ]. One of the first United States publications explicitly reporting the use of electronic computational methods is entitled the Electronic Energy of LiH and BeH + [ 354 ]. In a 1959 article entitled Broken Bottlenecks and the Future of Quantum Mechanics , Mulliken and Roothaan comment that a crucial step “is the programming for large electronic digital computers of the otherwise still excessively time-consuming numerical computation of these integrals, and of their combination to obtain the desired molecular wave functions and related molecular properties. The pioneering work in this field was that of Boys” and further report that “after much preliminary work in this Laboratory, we have developed a successful machine program for (a) computing accurately all the necessary integrals for diatomic molecules containing zeroth and first row atoms” [ 355 ]. This paper also shows the real impact that computers had on the field of computational chemistry as they further comment: “The importance of such a machine program is illustrated by the fact that the entire set of calculations on the N 2 molecule which took about a year, can now be repeated in 35 min, using a machine of moderately high capacity” [ 355 ].
With the greater availability of computational resources and commercial main-frames (and subsequently personal computers, clusters and super-computers), the area of computational chemistry blossomed to the present state, where complex calculations at the DFT or other levels (see the next section) can be carried out on a laptop using commercial programs.
5.2. Semi-Empirical Methods
One of the greatest influences of the availability of low-cost computational capacity was the acceptance by the chemical community that non-exact quantum chemical solutions could be of great benefit to the wider community. This resulted in the development of a whole range of semi-empirical methods, of which the Hückel model could be seen as the progenitor. Hoffmann further developed the Hückel approach into his extended Hückel method in which he considers not only the π-bonding but also the σ-bonding in the system [ 356 , 357 ].
As computational capacity became available, it was possible to return to methods that had been considered but rejected as calculationally too demanding in the past. The majority of the high-level calculations on multiatomic systems in the 1960s and 1970s are based on the Hartree–Fock self-consistent field method. This was first formulated by Hartree in 1927 (published 1928) as a method for calculating approximate wave functions and energies using ab initio methods [ 358 ]. The Hartree approach was criticized by Slater [ 359 ] and Fock [ 360 , 361 ] in terms of the antisymmetry of the wave function. Summarizing the Hartree–Fock approach, the Born–Oppenheimer approximation is obeyed and the nuclei are treated as static, relativistic effects are completely neglected and each energy eigenfunction is described by a single Slater determinant.
The semi-empirical methods attempt to address some of the deficiencies of the computationally demanding Hartree–Fock approximation for the determination of the stationary state wave function and energy of multi-body systems. They are driven by the competing desires on the one hand to simplify the computational demands, and on the other hand to increasing the speed and improving the accuracy of the calculations.
It is now time to enter into the world of acronyms by which many of these methods are known. These methods had their heyday in the last quarter of the 20th century C.E. but are still used when either a high level of accuracy or, paradoxically, computationally cheap and easy calculations are required. Most methods are based upon the neglect of diatomic differential overlap (NDDO), which allows simplification of the Hartree–Fock equations. A widely used implementation of the NDDO method was in the modified neglect of diatomic overlap (MNDO) approach introduced by Dewar in 1977 [ 362 ], but numerous related approaches including complete neglect of differential overlap (CNDO, 1965) [ 363 , 364 , 365 ] intermediate neglect of differential overlap (INDO, 1967) [ 366 ], modified intermediate neglect of differential overlap (MINDO, 1975) [ 367 ], and Zerner’s intermediate neglect of differential overlap (ZINDO, 1973) [ 368 ] were also developed.
Slightly different approaches were implemented in the Austin Model 1 (AM1, 1985) [ 369 ] model, which resembled MNDO but treated the nuclear-nuclear repulsion in a different manner and the parametric method 3 (PM3, 1989) [ 370 , 371 , 372 , 373 ], which is essentially a reparameterization of AM1.
5.3. Density Functional Theory
Today (2020), density functional theory (DFT) is one of the most common methods used for studying chemical systems [ 374 , 375 , 376 , 377 ]. The ab ibitio methods described above are still widely used and have advantages for some systems. However, and possibly more than any other advance in computational chemistry, DFT has made the synthetic chemist realize that quantum chemical methods are a tool as valuable in planning chemical transformations as spectroscopy and crystallography are in determining the nature of their products. The insight that DFT gives into chemical bonding is profound and has had a genuinely paradigm-shifting impact on chemistry. DFT is based upon the use of exchange-correlation functionals that use electron density to describe the many-body effects within a single particle formalism. At the core of the DFT method is the Hohenberg–Kohn theorem. The contribution of Walter Kohn was honored by the award of the 1998 Nobel Prize in Chemistry for the ‘‘development of the density-functional theory’’. The precise description of DFT as a calculational method is still debated, as is the degree and accuracy of the calculation of electron correlation [ 378 , 379 , 380 , 381 , 382 ].
For n -electron systems, exact quantum mechanical solutions can only be classically obtained for hydrogen atoms and helium monocations [ 383 ]. Hohenberg and Kohn proposed that the exact ground-state energy can be described as a function of a function (a functional) relating to the ground-state one-particle density [ 384 , 385 ]. All that remained was to develop appropriate functionals! Once these were available, the goal describing motions and pair correlations of a many-electron system by the total electron density became a seductive challenge. Kohn and Sham showed that ground state self-consistent equations could be developed that were analogous to the Hartree and Hartree–Fock descriptions. The exchange and correlation parts of the chemical potential of an electron gas are treated as additional effective potentials.
The model originated in physics and it was originally thought that it could not be applied to the detailed analysis of chemical systems at the atomistic level. However, relatively early in the development, it was shown that appropriate functionals gave good descriptions of the geometry and dissociation energy of molecules. Subsequently, the development of DFT allowed accurate prediction of energies along a reaction coordinate and the identification of reaction intermediates and transition states. Today, not only is DFT used to investigate the ground state properties of molecular systems, but also to study their excited state properties and the transition states and intermediates along a reaction coordinate. The increasing use of time-dependent DFT methods is allowing a profound insight into the behavior and properties of excited state species [ 386 , 387 , 388 , 389 ].
5.4. And Back to the Simplicity of Bonds—The Natural Bond Order Approach
Despite the success of molecular orbital theory, chemists were often left seeking the simplicity of the Lewis and valence bond descriptions of two-electron two-center bonds rather than the complexity of multi-center delocalized descriptions of bonding. In 1955, Löwdin introduced the concept of “natural orbitals” [ 390 ]. Natural bond orbital methods seek to represent electronic wavefunctions in terms of localized Lewis-like chemical bonds [ 391 , 392 ]. The computational method has been incorporated in many of the standard quantum chemical packages used in chemistry and analysis in terms of natural atomic orbitals, natural hybrid orbitals, natural bonding orbitals, and natural (semi-)localized molecular orbitals is common in some areas of chemistry. The approach has been adopted with various degrees of enthusiasm, although it appears very versatile and intuitive and has been extended to periodic systems, bulk solids, and surfaces [ 393 ]. Extension of the concept to hydrogen-bonding has proved to be more controversial [ 394 , 395 , 396 ].
One interesting development of the NBO approach is found in Boldyev’s adaptive natural density partitioning (AdNDP), which combines the simplicity of Lewis theory with the broader appeal of molecular orbital theory [ 397 ]. Chemical bonds are treated as n -centre 2-electron bonds, where n can vary from one (a lone-pair), through two (a conventional Lewis two-electron bond) to the maximum number of atoms in the system (completely delocalized bonding). This approach has found considerable success in describing the bonding in inorganic and main-group cluster compounds [ 398 , 399 ].
6. The Challenge Bonding
By the beginning of the 1960s, all was well in the chemical universe. Chemists understood and ordered materials according to the extreme descriptions of covalent two-electron two-center bonds and ionic charge-separated. Of course, delocalized bonding was understood and invoked when necessary and coordination compounds produced a conundrum falling somewhere between the two extremes. In the next half century, many of these conceptions and pre-conceptions were to be challenged, modified, discarded or replaced in the light of ever more interesting and challenging compounds being prepared and characterized.
6.1. Metals Bowl a Googly
In the language of the cricketer, just when you think you understand something, Nature has the tendency to bowl you a googly. The organic community had long accepted C–C multiple bonds, but inorganic chemists were blind-sided in the 1960s by the discovery of metal–metal multiple bonds [ 400 ]. How much of the initial skepticism was based in the memories of the Jørgensen chain theory is conjecture. Nevertheless, the metal–metal quadruple bond served to remind the community that metals still had a few tricks up their sleeves [ 401 ]. Some forty years later, the inorganic community was again surprised by compounds containing metal–metal quintuple bonds [ 402 , 403 , 404 , 405 , 406 ]. Even sextuple bonds have been identified in dimolybdenum (Mo 2 ) and ditungsten (W 2 ) [ 407 , 408 , 409 , 410 , 411 , 412 ]. These new types of bonds stimulated an enthusiastic and sometimes heated debate about the correlations between bond strength, bond order, and bond length. The novel The Delta Star by Joseph Wambaugh, is possibly the only work of fiction to feature Harry Gray and the spectroscopy of metal–metal multiply-bonded compounds [ 413 ].
6.2. When is a Bond not a Bond?
The modern chemist is confronted with an ever more complex and interesting array of types of bonding. There is an emerging and increasing interest in interactions that lie outside the classical Lewis two-electron two-center model, but which nevertheless find atoms closer together than their van der Waals radii would suggest.
The commonest example of this type would be the classical hydrogen bond, which is well-rationalized in models and calculational approaches involving multi-center bonding [ 414 ]. The concept of the classical hydrogen bonds dates back to Alfred Werner, who depicted ammonium chloride as NH 3 …HCl [ 415 ] and was revived by Latimer and Rodebush in their use of Lewis model to explain the anomalous properties of water [ 248 ]. The hydrogen bond was an integral part of the coherent views of molecular structure promulgated by Linus Pauling [ 316 , 317 , 318 ].
The increasing routine availability of crystallographic methods to characterize solid state materials, and in particular, the accessibility of databases and the use of artificial intelligence to analyze large sets of structural data, have allowed the identification and establishment of non-classical hydrogen bonds such as C–H…O, although these were first postulated in 1937 [ 416 , 417 ]. Once again, interactions of this nature have stimulated the debate about distance and energetics, although in most cases the “bond” energies are relatively low (4–5 kJ mol −1 ) [ 418 , 419 , 420 ].
Metals can also develop attachments to neighbors, and the term metallophilic was introduced in 1994 by Pekka Pykkö [ 421 ] as a general description of a phenomenon in which compounds with multiple metal centers exhibiting d 8 , d 10 , and s 2 outer shell configurations often show short (close to the sum of the atomic radii) contacts between metal centers, indicative of an attractive interaction rather than the expected repulsive ones. The description metallophilic was a general extension of the phenomenon of short Au(I)…Au(I) interactions in a large number of compounds and termed aurophilicity by Pykkö and others [ 422 , 423 , 424 , 425 , 426 , 427 ]. In gold compounds, the aurophilic interaction is typified by Au…Au distances close to 3 Å. Pykkö described the aurophilic interaction as amongst the strongest closed-shell interactions, with bond energies of 10–50 kJ mol −1 approaching those of weak covalent bonds or hydrogen bonds, although the word aurophilic was only coined in 1988 by Schmidbaur.
The general approach of investigating short interactions between molecules in the solid state has recently been described as the quantum theory of atoms in molecules (QTAIM) model [ 428 , 429 , 430 , 431 ]. QTAIM was originally seen as providing a physical justification for the Lewis bonding model and the pairing of electrons together with electron localization lie at the core of the description, but is evolving beyond that first vision. Bader, the originator of the QTAIM model, has also made the correlation with molecular orbital theory [ 432 ]. Closely related to QTAIM is the electron localization function (ELF), which is the probability of finding an electron in the neighborhood of a reference electron at a given point and possessing the same spin. The ELF not only shows a separation between the core and valence electrons according with chemical intuition, but also a visualization of covalent bonds and lone pairs [ 433 ].
It seems appropriate to end our scientific journey here, with methods that are beginning to provide a direct visualization of bonds.
7. Conclusions and Reflections
We have made a long journey from ancient Greece to modern bonding theory. It is certainly not a journey that has reached its end. We will finish our part of the journey with the words of Roald Hoffmann (as quoted by Philip Ball in an article entitled Beyond the Bond [ 434 ]): “Any rigorous definition of a chemical bond is bound to be impoverishing” and his advice to “have fun with the fuzzy richness of the idea”. Enjoy the fuzziness of the bond, think occasionally of the little hooks and do not let dogma or conventional views of bonding limit your creativity.
Acknowledgments
As always, it is a pleasure to thank the dedicated staff of the University Library in Basel who have assisted us in tracking down some of the more obscure materials. It is also a pleasure and an honor to thank all of those who have suffered from discussions on this topic or contributed valuable ideas and insights. We particularly thank the referees of this manuscript for their insightful comments and suggestions for further improvement.
Author Contributions
Writing—original draft preparation, E.C.C.; review and editing, C.E.H. All authors have read and agreed to the published version of the manuscript.
This research received no external funding.
Conflicts of Interest
The authors declare no conflict of interest.
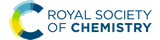
Teaching and learning chemical bonding: research-based evidence for misconceptions and conceptual difficulties experienced by students in upper secondary schools and the effect of an enriched text †

First published on 18th June 2018
Chemical bonding is a fundamental but complex topic, which has traditionally been associated with learning difficulties, misunderstandings, and misconceptions. This paper reviews some previous studies, concerning students’ conceptual difficulties and reports the findings from a research study with Greek students, which set out to examine their knowledge and understanding of a number of key concepts related to bonding. Three student samples were studied; one consisted of tenth-grade students from three public schools, the second contained first-year chemistry and biology students at the beginning of their university courses, and the third involved tenth-grade students from a prestigious private school. The students generally exhibited limited knowledge and possessed certain misconceptions, with the private school and the university students demonstrating better knowledge than the public school students. A quasi-experimental research design was employed using students from the private school, with some students used as a control group and others as a treatment group. The control group was taught using the standard Greek chemistry textbook, while the treatment group used enriched teaching material. It was found that while the two groups demonstrated similar performance for many bonding concepts, the treatment group did show superior knowledge with respect to a number of issues, such as the role of electrostatic interactions, electronegativity, and bond polarity.
Introduction
Student conceptual difficulties with chemical bonding.
The conceptual difficulties that students encounter when trying to understand chemical bonding lead many of them to resort to rote learning and the formation of numerous misconceptions ( e.g. Levy Nahum et al. , 2007 ), many of which prove resistant to instruction ( e.g. Nicoll, 2001 ; Taber and Coll, 2003 ; Özmen, 2004 ). It is perhaps not surprising then that the learning of chemical bonding has been the subject of many investigations, e.g. : Peterson et al. (1986, 1989) , Butts and Smith (1987) , Peterson and Treagust (1989) , Taber (1994, 1997, 1998) , Robinson (1998) , Birk and Kurtz (1999) , Tan and Treagust (1999) , Barker and Millar (2000) , Harrison and Treagust (2000) , Boo and Watson (2001) , Coll and Taylor (2001) , Coll and Treagust (2001, 2002) , Niaz (2001) , Nicoll (2001) , Taber and Coll (2003) , Özmen (2004) , Kronik et al. (2008) , Othman et al. (2008) and Ünal et al. (2010) . Ünal et al. (2006) carried out a detailed thematic review of chemical bonding studies by developing a matrix, which summarized their findings. The matrix incorporates the following themes: needs, aims, methods of exploring students’ conceptions, general knowledge claims, students’ alternative conceptions, implications and recommendations for teaching and learning, implications for curriculum development, and suggestions for future research.
It is well known that teachers rely on and closely follow textbooks in their everyday practice ( Britton et al. , 1993 ). However, when taught in the traditional way, the concept of chemical bonding can lead to misunderstandings by learners. Many of these misunderstandings result directly from the oversimplification of models used in student textbooks. In fact, misunderstandings can arise both from the presentation of a rather limited and sometimes incorrect picture of the issues related to chemical bonding and, also, because the way the topic is taught is often heavily influenced by the assessment procedures ( Levy Nahum et al. , 2010 ). Thus, the teachers’ main aim is often to prepare their students for future matriculation examinations. This can lead to a simplistic teaching approach ( Kronik et al. , 2008, Fig. 1, p. 1680 ), with superficial teaching, resulting in shallow learning and ultimately producing misconceptions and pseudo-conceptions.
In a seminal paper, based on an interview study with 16–19 year-old UK students, Taber (1998) proposed an ‘alternative conceptual framework’ for chemical bonding, according to which the ‘octet rule’ is used by the students as an explanatory framework for chemical stability and reactivity. Unfortunately, however, this can be responsible for “the development of a spread of ‘misconceptions’”. A common feature is that students adopt an anthropomorphic approach in their explanations of chemical bonding, thinking that atoms need or desire to fill their shells so that they obtain octets of outer electrons and acquire noble gas electronic configurations. ‡ The key characteristic of student thinking in this UK study was that species with ‘full’ valence electron shells (usually octets of electrons) have an inherent stability. This was seen as a driving force for chemical reactions, with reactions occurring to allow atoms to fill their shells. Taber (1999) considered this framework as a largely coherent theory-like basis for thinking about chemical stability, change and bonding (for a review, see Taber, 2013 ). In addition, he has provided both a theoretical background ( Taber, 2002a ) and classroom tools ( Taber, 2002b ) for diagnosing relevant student misconceptions and testing the popularity of the ideas elicited in the 1998 interview study. Focusing on student reasoning about chemical substances and reactions (including bonding), Talanquer (2013) maintains that in building their explanations, students fail to recognize leading causal agents or any other type of causal mechanism and demonstrate a cognitive bias toward teleological explanations, which are pervasive and resilient to conceptual change.
Following the paper by Levy Nahum et al. (2007) (see also: Kronik et al. , 2008 ), which developed “a new teaching approach (the ‘bottom up’ approach) for the chemical bonding concept aligned with current scientific and pedagogical knowledge”, there has been a revival of interest in teaching and learning of the topic of chemical bonding. Levy Nahum et al. (2013) have reviewed their work on chemical bonding.
Tsaparlis and Pappa (2012) examined fourteen general chemistry textbooks and identified similarities and differences with regard to the following aspects: presentation order of bonds (covalent and ionic bonds); placement and method of presentation of intermolecular bonds; the bond continuum; physical states and their relationship to the types of bonding; metallic bonding; the octet rule; electronegativity and bond polarity; and coordinate bonding. Almost all the books presented covalent and ionic bonding before intermolecular bonding, with a majority of books introducing the ionic bond before discussing covalent bonding. Most books refer to the covalent and ionic bonding continuum, though some continued to treat the two topics completely independently. Electronegativity and bond polarity are presented within covalent and ionic bonding, which most books seem to consider as the only true types of chemical bonding, with intermolecular bonds often being merely referred to as forces. While consideration of metallic bonding is included in the majority of these textbooks, many fail to even mention the coordinate bond. Finally, regarding intermolecular bonds, the books follow different orders of presentation, with most attention often given to hydrogen bonding.
Taber et al. (2012) used a diagnostic instrument, previously used in England ( Taber, 1994, 1997, 1998 ), to elicit the conceptions about bonding in NaCl (commonly used as the teaching example of an ionic compound) from two samples of students setting out on university courses in Greece and Turkey. The study reported that students in these two national contexts displayed high levels of support for statements based upon the alternative ‘molecular’ conceptual framework for thinking about ionic bonding identified in the English context, reinforcing the cross-national nature of the challenge to the effective teaching of abstract models of chemistry at the submicroscopic level. The study was later repeated in Croatia ( Vladušić et al. , 2016 ) and the findings were consistent with the previous ones.
Yayon et al. (2012) developed and tested a matrix, consisting of strands, to represent: (a) a systematic organization of the conceptual knowledge on chemical bonding required at high school level and (b) a tool for representing students’ conceptual knowledge of this topic. Using various instruments, students’ conceptual knowledge of chemical bonding was assessed and mapped onto the matrix, generating graphical representations of their knowledge. The matrix contains three strands: (i) the structure of matter at the nanoscopic level; (ii) electrostatic interactions between charged entities; and (iii) energy aspects related to bonding. Each strand consists of hierarchically ordered cells that contain fine grain elements of knowledge: the unbound atom; one bond between two atoms; a single molecule; many bonds of the same type (lattice); many bonds of different types; states of matter; overview. However, the matrix deals only with canonical knowledge, so misconceptions were not considered.
Bergqvist et al. (2013) analysed models of chemical bonding presented in chemistry textbooks at upper secondary level in Sweden and investigated themes in the textbooks which are related to students’ alternative conceptions and difficulties in understanding chemical bonding. The authors quoted examples of students’ alternative conceptions and difficulties in understanding for the different types of chemical bonding as these have been reported in the research literature, followed by possible sources of these difficulties according to the literature. The findings indicated that the models of chemical bonding represented in school textbooks are likely to be a cause of students’ alternative conceptions and difficulties in understanding. They therefore, suggested that there was a need to close the gap between textbook writers and current educational research.
Wang and Barrow (2013) carried out a comparison of the conceptual frameworks of chemical bonding possessed by undergraduate general chemistry students with high and low levels of content knowledge. It was found that high content knowledge students viewed chemical bonding as an electrostatic force between two atoms and justified bond formation with the octet rule and stability; in addition, they were comfortable with exceptions to the octet rule, and associated bond polarity with differences in electronegativity. On the other hand, low content knowledge students viewed chemical bonding as some type of (attractive) force between two atoms and justified bond formation with the octet rule and stability or with teleological explanations. They tended to be uncomfortable with exceptions to the octet rule and to possess misconceptions, but, like the high content knowledge students, they associated bond polarity with differences in electronegativity.
Hazzi and Dumon (2014) examined the extent to which Algerian undergraduate students were able to integrate the Lewis and quantum models of atoms and molecules. It was found that the students were not able to correctly describe covalent bonds in a Lewis structure using the concepts of the quantum model. According to the authors, students’ conceptions of atomic or hybrid orbitals act as a pedagogical impediment to learning, so the students use the electron pair framework, which is based on the use of Lewis model paired valence electrons, to form covalent bonds. In addition, the students believed that a covalent bond arises from the sharing of one electron (either s or p but not sp n ) from each atom to give one σ electron pair, and thought that σ bonding occurs only in single bonds.
Luxford and Bretz (2014) conducted interviews with 28 high school physical science and chemistry students, and general chemistry university students in order to investigate their understanding and misconceptions about covalent and ionic bonding representations through analysis of both student-created and expert-generated multiple representations used to communicate bonding concepts such as Lewis structures, formulas, space-filling models, and 3D manipulatives. Misconceptions were identified regarding four themes: (i) periodic trends; (ii) electrostatic interactions; (iii) the octet rule; and (iv) surface features associated with the representations (for instance, lines indicate bonds or spacing of the dots between atoms indicates equal sharing). Furthermore, a concept inventory, the Bonding Representations Inventory (BRI), was developed to quantify the prevalence of these misconceptions. The BRI was administered to 1072 high school chemistry, advanced placement chemistry, and general chemistry students across the United States, and content validity, concurrent validity, and reliability of the instrument were determined. The BRI was subsequently tested successfully in the Slovak educational system, where misconceptions were identified, and the results were compared with those in the USA ( Vrabec and Prokŝa, 2016 ).
Burrows and Reid Mooring (2015) used concept mapping to uncover undergraduate general chemistry students’ knowledge structures about chemical bonding concepts and detected several common misconceptions concerning electronegativity, with a weaker understanding of electronegativity among students with low concept map scores than for students with high concept map scores. Further, a lack of understanding of electronegativity led to a misunderstanding of polar covalent bonding.
Cheng and Oon (2016) developed an instrument and carried out a very large ( n = 3006) survey of year 10–12 students on their understanding of metallic bonding. The instrument probed into students’ understanding of metallic bonding as: (a) a submicro structure of metals; (b) a process in which individual metal atoms lose their outermost shell electrons to form a ‘sea of electrons’ and octet metal cations; and (c) an all directional electrostatic force between delocalized electrons and metal cations. According to the findings, the structure, process and interaction understandings were unidimensional and in an increasing order of difficulty. The instrument also assessed students’ explanations for the malleability of metals.
Nimmermark et al. (2016) carried out a large study with almost 700 Swedish and South African chemistry students from upper secondary school and first-term university levels, and reported that only about 20% of students had a clear grasp of the concept of bond energetics on coming to university, often confusing individual bond formation and breakage with exo- and endothermic processes. Also, an over-use of ball and stick models can lead to students having difficulty with visualization of the correct shape of molecules. Students who had encountered the VSEPR model in secondary school tended to have a better grasp of molecular shapes. It was also reported that an understanding of the quantum mechanical model of bonding was beneficial to undergraduate students.
Ballester Pérez et al. (2017) investigated Spanish students’ understanding of chemical bonding and their related misconceptions. The students came from secondary schools and from first-year undergraduate chemistry and pharmacy courses, while the topics studied included the interpretation of some properties of substances (colour, boiling points, solubility and conductivity), intra- and intermolecular forces, hydrogen bonding, covalent and molecular networks, geometry and polarity of molecules. The main misconceptions were: attributing macroscopic properties to particles; incorrect prediction of boiling points; perceiving ionic compounds as being formed by molecules; misunderstanding the nature of the hydrogen bond and assuming that it is present in any molecule containing hydrogen together with nitrogen, oxygen or fluorine, regardless of whether the hydrogen atom is directly bonded to these atoms or not; confusing the geometry of a molecule with the distribution of electron pairs around the central atom; and predicting the polarity of molecules incorrectly.
In conclusion, the prevailing approach to teaching chemical bonding continues to lead to learning difficulties and misunderstandings for many students. The following are some typical examples:
(a) The ionic bond is referred to as just a transfer of electrons between separate atoms in order to acquire full valence shells.
(b) The model of the covalent bond is described as an electron-pair that is shared between two atoms.
(c) The octet rule is often used by the students as an explanatory framework for chemical stability and as a prerequisite for a ‘proper’ bond.
(d) Covalent and ionic bonds are often presented in isolation, as bonds that share and bonds that transfer electrons respectively.
(e) Bond polarity is directly linked to the covalent bond.
(f) Covalent and ionic bonds are described as ‘real’ chemical bonds, while most intermolecular bonds are simply referred to as forces.
The present study
(1) To what extent do Greek upper secondary school students share a number of common misunderstandings and misconceptions about chemical bonding?
(2) What is the effect of an enriched educational text, based on the standard Greek chemistry textbook, on preventing the various misunderstandings and misconceptions in the case of high-achieving, tenth-grade students?
In a follow-up publication to this work, we will consider a spiral curriculum and learning progressions in the teaching of chemical bonding, taking into account recommendations from the research literature and our findings/answers to the two research questions posed above.
Educational material
The treatment group (TG) was taught using a modified/enriched text, which was based on the standard text, had the same format, and included the same exercises and problems at the end, but only for the topic of chemical bonding. This material was written by one of the authors (GT), who took into account, on the one hand the education research literature on the topic of chemical bonding, and on the other hand his personal scientific knowledge of this topic. Special attention was paid to the findings of the Tsaparlis and Pappa (2012) study of the way in which general chemistry textbooks presented the topic of chemical bonding (see above).
Two chemistry teachers had previously trialled an initial version of the material with their tenth grade classes, and provided feedback about its strengths and weaknesses. Their feedback was taken into account in writing a second version, which was subsequently reviewed by the two teachers and by a third chemistry teacher. The comments of these three reviewers were used in revising the material to provide the final text, which we label as the ‘enriched text’. Each student in the TG was supplied with a booklet containing a printed copy in colour of this enriched text (in Greek). However, these students also had available the standard textbook, so they could readily consult and compare both texts. See Appendix 2 (ESI † ) for a reproduction of the enriched text in English. The translation from Greek into English was again carried out by one author (GT) and checked by another (BB).
Regarding the basic features and differences between the standard and enriched texts, we should first acknowledge that the enriched text involves a considerable lengthening from 10 pages in the original Greek text, not including exercises and problems (9 pages in the English version, see Appendix 1, ESI † ), to about 15 pages (both in Greek and in English), that is, an increase by a factor ca. 1½. We also note the following in brief (see Appendix 3 (ESI † ) for a detailed comparison):
• Electrostatic interactions : The standard text does not mention anywhere the term ‘electrostatic interactions’, but it refers to electrostatic Coulomb forces. The enriched text also does NOT refer to ‘electrostatic interactions’ but does mention ‘Coulomb's law’, ‘forces of electrostatic nature (Coulomb forces)’, and ‘Coulombic interactions’.
• Octet rule : The enriched text includes a more detailed discussion about the octet rule, with emphasis on the fact that the octet rule does not “impose” the formation of an ionic bond, but is able to explain the charges on the ions and the stoichiometry of ionic compounds.
• Electronegativity . The enriched text gives a more detailed coverage, including the use of a quantitative scale (the Pauling scale) to this topic, and its contribution to the understanding of the continuum between ionic and covalent bonding.
• Bond polarity . While the standard text does not use the term ‘bond polarity’ or ‘polarity’, the enriched text refers explicitly to this concept. Both texts, however, refer extensively to nonpolar and polar bonds.
Type of questions
Pearson and Johnson (1978) proposed a classification that is particularly suitable for the evaluation of questions (see also Pappa and Tsaparlis, 2011 ). Their classification refers to three kinds of knowledge: declarative knowledge, procedural knowledge, and metacognition. Declarative knowledge is knowledge of what, while procedural knowledge is knowledge of how. Procedural knowledge involves higher-level learning, requiring justification for the preference of one process instead of another, the making of hypotheses, observation, recording of data, and arriving at conclusions. Finally, metacognition aims to identify the extent to which the student has acquired autonomous thinking and action ( Schneider, 2004 ).
Pappa and Tsaparlis (2011) evaluated the questions contained in the chapters on chemical bonding of a number of general chemistry textbooks, and reported that closed-type questions, by far, outnumbered the open-type ones. Questions were mainly of the ‘short answer’ type, with the majority testing for declarative knowledge and fewer questions testing for procedural knowledge. A complete lack of metacognitive questions was found.
Research instrument
The test consisted of two parts. The 1st part had to be answered first, and gave the students a list of concepts that they had to identify according to whether:
• they are used or not for the description of bonds, and if so:
• what type or types of chemical bond they describe.
Next for each term, the students were asked to assign one of the following symbols:
• ‘IB’, if the term is used to describe the ionic bond only;
• ‘CB’, if the term is used to describe the covalent bond only;
• ‘IB&OB’, if the term is used to describe both ionic and covalent bonds;
• ∅, if the term is used to describe NEITHER ionic NOR covalent bonds;
• N/A: I don’t answer.
The concepts given to the pupils are listed in Table 1 .
The 1st part of the test was completed by asking students to use concepts listed in Table 1 to write up to two sentences for each of the following cases: (i) relating exclusively to the covalent bond, (ii) relating exclusively to the ionic bond, and (iii) relating to both types of bonds. A 20 minute timespan was allowed for completing the 1st part of the test.
In the 2nd part of the test, learners were given four questions (see Table 2 ). The first question entailed six disjunctive (Right/Wrong) statements about basic concepts regarding the chemical bond; in addition, students had to justify their answers. The second question concerned the criteria used for determining the type of bond. The six concepts of question 1 together with question 2 provide the seven items that are examined in Part 2 of the test of the current paper. Finally, there were two questions of the metacognitive type (questions 3 and 4 in Table 2 ); these required students to state the difficulties they had experienced in connection with the study of chemical bonds. A 20 minute timespan was also allowed for completing the 2nd part of the test.
The level of justifying answers was evaluated using the SOLO taxonomy ( Biggs and Collins, 1982 ; Biggs, 1999 ), which distinguishes among five levels of understanding: (1) prestructural, (2) unistructural, (3) multistructural, (4) relational, and (5) extended abstract. We will report the findings from this analysis in another publication, but suffice to comment here that the justifications offered by most students for their choices were at the lowest two levels (prestructural, and unistructural) of the taxonomy. Only for one question (that on the criteria for bond-type formation, which just required the enumeration of a number of pieces of knowledge) were there performances that exceeded the second level, while just nine students from among the 46 high-achieving students of the treatment group achieved an average over the second SOLO level. Few answers reached the third (multistructural) level.
Ethical considerations
Student samples.
The rationale for including the two university departments in our study was as follows. The students were at the very beginning of their university studies, so their knowledge about chemical bonding derived from their overall studies in secondary education. Because of the subjects of their study (chemistry and biology), all these students had studied chemistry as an advanced course and chemistry was among the subjects examined in their matriculation examinations.
Before proceeding, it is also essential to explain why we have treated, for convenience, the three public schools as a single sample and the two university departments as a single sample. There was a large variation in individual performance for students at the public schools. This could be due (at least in part) to random factors (such as guessing) plus the added fact that we are dealing with small samples ( n = 22) from each school. The averaged performances for each school over the seven items of the 2nd part of the test of this study were found to be 34.4, 44.8, and 46.1%, showing a similar overall performance for the second and third schools, with the first school lagging behind. So our treatment of the three schools as a single group (PuS, n = 66) can be justified by the fact that they had the lowest performances among our three samples, but, in any case, our sampling frame should not be assumed to be representative of the average Greek public school.
In the case of the students from the two university departments, there was again a variation of performances which could also be due (at least in part) to random factors [in addition, the samples ( n = 34 and 38 respectively) were again not large]. However, the average performance for each sample in the seven items was about the same: 55.9% for CHE and 57.5% for BIO. For this reason and for convenience, we combined the two university departments into a single group (university students, US). Note that the corresponding average (54.3%) for the control group from the private school (see below) is similar to that for the university students (56.8%). We have not combined these two samples (US and PrS), both because they correspond to quite different populations in terms of origin and age and also because of the differing demands for knowledge retention: the school students were tested on completion of the teaching, whereas the university students had received their teaching some considerable time before testing.
In the case of the tenth-grade students from the private school, the survey was conducted in two parts: in school year 2012–2013 for the control group [PrS(CG) or simply CG, n = 49]; and in school year 2015–2016 for the treatment group [PrS(TG) or simply TG, n = 46]. The teacher had a master's degree in chemistry education and new educational technologies, had taught at this particular school for sixteen years, and in addition had five years of experience as a private tutor. Students in the CG were taught the topic of chemical bonding using the standard textbook (see Appendix 1, ESI † ), while students in the TG were taught this topic making use of the enriched text (see Appendix 2, ESI † ).
At this point, it is important to explain exactly what we mean by the term ‘prestigious private school’. The school in question has outstanding facilities including laboratories, is renowned for the quality of both students and teachers, and provides a range of extracurricular activities. Because, of the tuition fees, the school draws its students from relatively high-income families. We provide above, in Table 3 , data for the coursework, final examination and cumulative yearly student evaluation in the chemistry course, and the overall average yearly mark achieved for all courses, to demonstrate the quality of students at this private school. Table 3 includes school performance data for the students at the school for both CG and TG samples. Note the lower marks in the final end-of-year written examination in chemistry, compared to those for continuous assessment based on overall course participation, including oral and written test performance.
Table 3 considers two samples of students for both the CG and the TG: the total sample ( n = 53 and 46 respectively), || and a reduced sample ( n = 47 and 33 respectively), in which the 6 and 13 students respectively with a cumulative yearly mean mark in chemistry below 80% have been excluded. We observe that 89% of the students in the case of the CG and 72% in the case of the TG were high achievers, while achievements by the remaining students (more students in the case of the TG) were more modest.
Comparison of the results obtained by the CG and TG (whole samples) by means of the statistic t for independent samples (two-tailed tests), using the separate variances formula, gives the following values: for the chemistry continuous assessment, t = 1.29, which is not statistically significant; for the final chemistry examination, t = 2.90, which is significant at the 0.01 level; and for the cumulative chemistry mark, t = 2.49, which is statistically significant at the 0.05 and 0.02 significance levels but not at the 0.01 level; finally, for the overall yearly mean mark, t = 1.35, which is not significant.
Note that it is results in the chemistry final examination, which have resulted in a significant difference in favour of the CG, but the difference might not be due to any actual difference in teaching between the CG and the TG but could reflect more demanding exam questions in 2015–2016 compared to 2012–2013. The two PrS samples (the CG and the TG) can be considered as more or less equivalent for a number of other reasons: (i) they were from the same school; (ii) the selection method and the method of awarding bursaries to part of the students were the same, (iii) they had the same chemistry teacher, (iv) they were chosen randomly from the tenth-grade populations of school years 2012–2013 and 2015–2016 respectively; and (v) no changes in the curriculum, the chemistry textbook or the school programme occurred between 2012–2013 and 2015–2016. In any case, the possibility that the CG had a small superiority with respect to the TG can only serve to strengthen our findings from the comparison between the CG and the TG.
Results and discussion: 1st part of the assessment instrument
1st part of the assessment instrument, all samples.
• Concepts that refer to tautologies (chemical bond, ionic bond, covalent bond, ionic compound, covalent compound).
• Concepts that have no relevance to chemical bonding (mass, volume, density, percentage amount).
• Concepts that are directly relevant to bond-type and bond formation ( e.g. energy, electronegativity, octet rule).
• Concepts that are only indirectly relevant to bond-type and bond formation ( e.g. mass number, metal, non-metal, solubility).
Knowledge of the first two classes was used to test the equivalence of the control and treatment groups and the general reliability of our data.
Abstentions : Examining the frequency of the option N/A (I don't answer), we note that the abstentions varied considerably from topic to topic but overall levels were disappointingly high, suggesting a lack of understanding. BIO and TG students seem to use this option the least, with frequencies <10%, while levels for CHE were high. PuS students also exhibited several high rates of abstention.
Knowledge of tautological concepts (chemical bond, ionic bond, covalent bond, ionic compound, covalent compound): With the exception of the PuS in the case of the chemical bond concept, correct responses ranged from 84 to 100%, with 17 out of the 20 cases being above 90%. In the case of the chemical bond concept (where as correct we assumed the IB&CB option), PuS had only 45% correct answers, with 22% opting for the ∅ answer, that is, the term ‘chemical bond’ is neither used to describe ionic nor covalent bonds. This finding shows that for many students the adjective ‘chemical’ differentiated a bond from both ‘ionic’ and ‘covalent’ bonds, and demonstrates the overall lower level of knowledge of the PuS students.
Knowledge of concepts having no relevance to chemical bonding (mass, volume, density, percentage amount). Frequencies of the expected ∅ choice ranged from 65 to almost 90% (96% for TG, with the PuS having the lowest frequencies).
Knowledge of concepts directly relevant to bond formation Table 4a gives the results for the first part of the test for knowledge of the concepts directly relevant to bond-type and bond formation.
Ion. The expected answer for this concept was that it is relevant to IB only. However, for PuS and CG less than 60% of the students provided the correct answer. Considerable percentages (>20%) of answers favoured the option IB&CB in all cases (except for TG: 13.0%). The TG had the highest percentage correct answers (80.4%). It seems likely that the idea that a polar covalent bond possesses some ionic character was the cause of these results.
Crystal. The expected answer for this concept was that it is relevant to IB only. (Of course, crystals do exist in the case of covalent compounds too, but this issue was not included in our lessons.) PuS and US had relatively low frequencies (close to 70%), while both PrS samples had the highest frequencies of correct answers (≈90%).
Molecule. The expected answer for this concept was that it was relevant to CB only. However, the responses from both US (34.7%) and PuS (43.9%) were disappointing. Better results were found in the cases of CG (63.3%) and TG (84.4%).
Metal . The expected answer for this concept was that it was relevant to IB only. However, a high percentage of correct answers was found only for CG (83.7%) and TG (82.6%); lower frequencies of correct answer were found for the other two samples, with IB&CB providing relatively high percentages of choices. (Of course, there are many examples of metals being involved in covalent bonding e.g. aluminium trichloride and a variety of organometallic compounds, but these had not been introduced into the teaching at this stage.)
Nonmetal . The expected answer for this concept was that it was relevant to both IB and CB but low percentages of the correct choice were found, in all cases. This disappointing finding is not easy to explain but the issue clearly needs to be addressed in the teaching.
Electrostatic forces. Table 4b gives the results for the first part of the test for entries about electrostatic forces that are relevant to bond-type and bond formation.
Coulomb forces. The expected answer occurred with a high frequency only for the TG (87.0%), while for all other samples, frequencies were very low. Many students associated these forces with IB only, showing the connection with the attractions between ions. Indeed, the standard text discusses these forces only in connection with the IB. Frequencies of the ∅ option were disturbingly high.
Attractive forces. Here we found high levels of the correct answer, especially for US and TG.
Repulsive forces . Surprisingly, here we find very low percentages of the correct answer, in all cases. The high frequency of the ∅ option is noteworthy. It would appear that most students associate the concept of bonding only with attractive forces, and therefore ignore the repulsive forces (electron–electron and nucleus–nucleus repulsions). Clearly, this finding suggests that this likely misconception needs to be carefully considered when teaching chemical bonding.
It should be noted that both the standard school textbook and our enriched text contain regular references to Coulomb forces/Coulomb interactions, with repulsive forces being mentioned to a lesser extent. Nonetheless, the school textbook does contain an explicit reference to repulsive forces, at one point: “A chemical bond is created when the structural units of matter (atoms, molecules, or ions) come close enough together, so that the attractive forces that are developed between them ( e.g. , between the nucleus of one atom and the electrons of another atom) overcome the repulsive forces involved ( i.e. between the nuclei and between their electrons)” (p. 1). Although the enriched text does not include such a reference, when considering a crystal lattice it is explicitly stated that “oppositely charged ions will attract each other, while ions with charges of the same sign will repel each other, through Coulombic interactions” (p. 7).
Table 4c lists the results for knowledge of other concepts directly relevant to bond formation: energy, valence electrons, the octet rule, electronegativity, polarity, and Lewis structures.
Energy: With the exception of PuS (where only 29.8% of the students chose the correct option and 50.8% chose ∅), frequencies were good with US and CG (≈70%), and the TG achieving the highest percentage of correct answers (82.6%).
Valence electrons : Performance here was moderate, with only about 55% correct answer for PuS and US, but much higher (near 90%) for the two PrS samples.
Octet rule . Results are similar to those for valence electrons (see above).
Electronegativity . Results were poor to moderate, except for TG, who recorded an excellent percentage of correct answers (95.7).
Polarity . Results demonstrated similar trends to those for electronegativity (see above).
Lewis structures . With the exception of PuS, where we found a very low frequency of correct answers (28.4%, with 52.2% opting for the CB only response), the other results were satisfactory, though not high, except for CG (87.8%).
A similar pattern of results, showing low frequencies of correct answers (and considerable percentages choosing the option ∅), is found, with both PuS and US. The results are similar for the CG in some cases, but those for the TG are generally satisfactory (>69%).
Sentences composed by the students about chemical bonding
Comparison of the control and treatment groups from the private school for the 1st part of the assessment instrument.
The remaining nine concepts in Table 7 are relevant to the description of both types of bond. Data for the concepts of ‘energy’, ‘valence electrons’, ‘octet rule’, and ‘Lewis structures’, reveal only none statistically significant differences between CG and TG. Results for the concepts of ‘electronegativity’, ‘polarity’, and ‘Coulomb forces’ are of more interest: the TG outperformed the CG, with the TG frequencies exceeding 80% and differences being statistically significant. This finding is consistent with the fact, that, while the enriched text emphasized this property for these concepts, the standard textbook did not. The CG students provided a very low percentage of correct answers for both ‘polarity’ (12.2%) and ‘Coulomb forces’ (8.2%). The majority of CG students (65.3%) associated the concept of ‘polarity’ only with the covalent bond, while in the case of ‘Coulomb forces’ the majority of students (61.2%) associated the concept only with the ionic bond. The fact that the standard text discusses polarity only in connection with the covalent bond and Coulomb forces only in connection with the ionic bond probably explains these findings. On the other hand, ‘attractive forces’ were used to discuss both types of bonds for both the CG and the TG, and percentages of correct answers were high for both groups (71.4% for CG and 91.3% for TG, with the difference being statistically significant at the 0.05 level).
Results and discussion: 2nd part of the assessment instrument
2nd part of the assessment instrument, all samples.
Considering performances in the individual questions suggests that the question on the octet rule was by far the most difficult for all four of the samples, while the questions on ‘electrostatic interactions’ and on ‘bond polarity’ also proved difficult. The questions on the ‘criteria for bond-type formation’, the ‘valence electrons’ and the ‘Lewis structures’, on the other hand, seem to have been relatively easy. Specific comments about these findings are given below, with reference, where appropriate, to the contents of the standard and enriched texts. The basic features and differences of the two texts were described in subsection ‘Educational material’, but for a detailed comparison, see Appendix 3 (ESI † ).
Electrostatic interactions : The question concerning electrostatic interactions produced poor performances, except for the TG. The standard text does not mention anywhere the term ‘electrostatic interactions’, but it does refer to ‘ forces of an electrostatic nature/Coulomb forces’. The enriched text also did NOT refer to ‘electrostatic interactions’, but referred to ‘Coulomb's law’, ‘forces of electrostatic nature (Coulomb forces)’, and to ‘Coulombic interactions’.
Valence electrons : Good performances were found for the first three samples, while the performance by the TG was very good.
Octet rule : Here we found a very small percentage of correct answers for the role of the octet rule, even for the TG. The standard textbook contained relatively little relevant information compared with the enriched text. It is therefore surprising and disappointing that even the students of the TG performed so badly in this question.
Electronegativity : The first three samples had relatively low performances (44, 56, 71% respectively), but the TG performed very well (91%). These findings can be justified by comparing the coverage of electronegativity in the standard and the enriched texts, with the latter giving a more detailed coverage, including the use of a quantitative scale (the Pauling scale), for this property. The enriched text discusses electronegativity with reference to nonpolar covalent bonding, nonpolar and polar covalent bonds, the ionic bond, the continuum between ionic and covalent bonding, and the percentage ionic and covalent character of a bond.
Bond polarity : The findings are similar to those for the electronegativity concept, with the first three samples having low performances (36, 40, and 18% respectively) and the TG giving a very high performance (91%, the same as for electronegativity). It is noteworthy that the standard text does not use the term ‘bond polarity’ or ‘polarity’, while the enriched text refers explicitly to this concept. Both texts, however, refer extensively to nonpolar and polar bonds. Of particular relevance is the following statement in the enriched text in relation to polar covalent and ionic bonds:
“Chemical bonds between atoms of different elements are polar, with a partially ionic and a partially covalent character. If the covalent character is dominant, we have a polar covalent bond , if the ionic character dominates, we have ionic bonding ”. (p. 15)
Lewis structures: With the exception of the public schools (30%), all other samples had high performances (83, 100, 98%).
Criteria for bond-type formation: Public schools were again an exception, with a relatively low performance (54%), while all other samples performed well (92, 90, 96%).
Comparison of the control and treatment groups from the private school for the 2nd part of the assessment instrument
Performances in the 2nd part of the assessment instrument for the reduced sample and the remaining students from the treatment group, metacognitive questions and students’ opinions about the enriched text, conclusions and the way forward.
Research question (1): To what extent do Greek upper secondary school students share a number of common misunderstandings and misconceptions about chemical bonding?
List of identified misconceptions and conceptual difficulties
• A considerable number of PuS students thought that a ‘chemical bond’ was different from either IB or CB (that is, the adjective ‘chemical’ was thought to differentiate a bond from both IB and CB).
• The idea that a polar covalent bond possesses some ionic character leads to the misconception that ‘ions’ are involved in both IB and CB
• The idea that the concept of ‘molecule’ is relevant to both IB and CB (and not only to CB) is widespread. The relevancy of the concept of ‘metal’ to IB only was not universally accepted.
• Few students recognized that the concept of ‘nonmetal’ was relevant to both types of bond.
• While students appeared to have little difficulty accepting that ‘attractive forces’ were involved in both types of bonding, many students associated Coulomb forces with IB only.
• A majority of students appeared to believe that ‘repulsive forces’ are not relevant to chemical bonding.
• A large majority of students were not only wrong in believing that the ‘octet rule’ could be used to explaining chemical stability and reactivity, but also mistakenly assumed that it could be used to predict whether atoms will form ionic or covalent bonds.
• The direct connection of the concepts of ‘electronegativity’ and ‘bond polarity’ to both IB and CB was not universally recognized, though students in general accepted that ‘Lewis structures’ were of relevance to both types of bond.
• Many students failed to recognize any connection between the concepts of ‘group’, ‘period’, ‘melting point’, ‘boiling point’, and ‘solubility’ to chemical bonding.
In general, weaknesses were evident for a large number of the examined concepts, especially in the case of the public school students (PuS). Only in the cases of ‘criteria for bond formation’, ‘valence electrons’ and ‘Lewis structures’ did the concepts appear to be well understood and even here the performances of the PuS students were limited. There is clearly much room for improvement.
Comparing our findings with those of previous studies, we note that the key feature of student thinking in the UK study by Taber (1998) that a ‘full’ valence electron shells (usually octets of electrons) gives atoms stability was apparent in our samples too. Wang and Barrow (2013) reported a similar finding. Indeed the ‘octet rule’ clearly remains a major source of student misconceptions. Luxford and Bretz (2014) identified misconceptions about periodic trends, electrostatic interactions, and the octet rule in their study. On the other hand, Burrows and Reid Mooring (2015) noted a lack of understanding of electronegativity and misunderstanding of polar covalent bonding. Finally, incorrect predictions regarding boiling points and the polarity of molecules were among the findings reported in the study by Ballester Pérez et al. (2017) .
Remarkably, in many cases, the control group (CG) from the PrS showed similar results to those obtained by the university students. This can be justified by the fact that we are considering a prestigious high school, with high-level students and high quality teaching, while the university freshmen represented a specific group with a longer exposure to chemistry over all three upper secondary grades.
Focusing attention on the 1st part of our assessment instrument (the ‘test’), we note that for many PuS students the adjective ‘chemical’ appeared to differentiate a bond from ‘ionic’ and ‘covalent’ bonds. The concept of ionic character for a polar covalent bond seems likely to have led many students to select the option IB&CB. Only the TG students from the private school appeared to appreciate the importance of Coulomb forces to both types of bonding. Many students only associated these forces with ionic bonding, apparently because of the attractions between ions. The standard textbook only discusses these forces in connection with the ionic bond, and this seems likely to be responsible for this finding. Knowledge of repulsive forces, which many students failed to link with chemical bonding, was very disappointing.
In contrast, knowledge of attractive forces, which most respondents correctly associated with both types of bond, was good. Electronegativity, polarity and Lewis structures (the last not by the PrS students), were assumed by many students to be of relevance only to covalent compounds. Finally, when considering knowledge of concepts that are only indirectly relevant to bond formation, we found low frequencies of correct choices (and correspondingly significant percentages opting for the ∅ choice), for group, period, melting point, and boiling point. However, the TG students provided satisfactory percentages of correct answers for all concepts, except solubility.
Research question (2) : What is the effect of an enriched educational text, based on the standard Greek chemistry textbook, on preventing the various misunderstandings and misconceptions in the case of high-achieving, tenth-grade students?
The quasi-experimental research design of the 1st part of the test, which sought to test knowledge of many concepts that relate directly to bond formation, detected no statistically significant differences between the control group (CG) and the treatment group (TG) for crystal, metal, energy, valence electrons, the octet rule, and Lewis structures. On the other hand, statistically significant differences in knowledge were detected for the concepts: molecule, non-metal, electronegativity, polarity and types of electrostatic forces (Coulomb, repulsive and attractive forces, although for the final concept performance was very low for all samples).
The findings for the concepts of electronegativity, bond polarity, and Coulomb forces were consistent with the fact that the enriched text places emphasis on the fact that these concepts relate to both types of bonding, while the standard textbook fails to do this. Consequently, the majority of CG students associated polarity only with covalent bonding and Coulomb forces only with ionic bonding. Considering concepts that are only indirectly relevant to bond formation, TG students demonstrated a superior knowledge of the concepts of period, mass number, atomic radius, and solubility, but not of the concepts of group, melting point and boiling point.
Turning to the 2nd part of the test, we note that the question about electrostatic interactions resulted in low performances, except for the TG. Very small percentages of correct answers were found for the role of the octet rule in bond formation, even for the TG, despite the fact that the modified/enriched text clearly indicated the limitations of the octet rule when discussing bonding. It would appear that the ‘octet framework’ is not only being used as a heuristic device, but is also serving a teleological function, with students regularly but incorrectly seeking to use it as an explanatory tool ( Talanquer, 2007 ).
Very high levels of correct answers (91%) were found with the TG for the concepts of electronegativity and bond polarity, with much poorer performances being found for the other three groups. These findings can be justified by comparing the coverage of the concepts in the standard and the enriched texts, with the latter giving a much more extended coverage. Finally, for Lewis structures and criteria for bond-type formation, performances were good except for the PuS students.
In conclusion, the TG produced a statistically significantly superior performance for the questions on electrostatic interactions, electronegativity, and bond polarity, though no significant differences were found in the cases of valence electrons, the octet rule, Lewis structures or criteria for bond formation. The extent of the problem with the octet rule and its resistance to change is a major significant finding of this study: students tend to treat the octet rule as a simple predictive algorithm even when the teaching specifically contradicts this approach, as was the case with the TG.
Final remarks
The question we must ask ourselves is, can the role of the modified/enriched text be considered advantageous in dealing with fundamental student misconceptions concerning chemical bonding? While the answer could be yes, it could also be no, especially when we take into account that the intervention was carried out with a prestigious private school, whose students demonstrated high performances in school courses, including chemistry. One might well expect lower performances and higher persistence of misconceptions with less able students.
Understanding the topic of chemical bonding requires the appreciation of many critical details and some sophisticated reasoning, making it complicated and therefore difficult for a large majority of the general student population. In a subsequent publication, we will present data derived from the two metacognitive questions given to the treatment group of students from the private school. These data represent the opinions of students towards the topic as it was presented in the enriched text, and highlight a serious problem, revealing that over one third of the students expressed negative comments about a number of issues (too lengthy; too dense; too complicated; with a lot of details; a lot of definitions; a lot of examples). Content overload is indeed recognized to be a major problem in modern chemical education, leading to curricula that “are too often aggregations of isolated facts” and to the students lacking “a sense of why they should learn the required material” ( Gilbert, 2006, pp. 957–958 ). This data, coupled with the supporting research-based evidence presented here lays a foundation for consideration of a spiral curriculum and of learning progressions in the teaching of chemical bonding. In a subsequent publication, we will look to review earlier studies which made proposals about the teaching of the topic of chemical bonding, and will provide our own proposals, which include defining lower, intermediate, and upper anchors of relevant scientific knowledge.
We would like to finish by considering a number of important issues that were raised by a reviewer of our original manuscript. In the design of the new learning materials, consideration should be given to the following:
• The principles underlining chemical bonding should be stated as basic ideas of our models, rather than as chemical facts. For instance, in the exercises and problems (pp. 10–12 of the standard text – see Appendix 1, ESI † ), there is no sense of modelling, with ideas being presented merely as facts. On the other hand, it was recognized that there is occasional reference to modelling in the enriched text.
• Care should be taken to prevent common textbook- and teacher-generated misconceptions. For instance, the standard Greek textbook presents (both in the text and in the exercises), the formation of ionic compounds by electron transfer between single atoms (and this is also followed by the enriched text), leading to the common misconception of representing ionic compounds as molecules. This usual practice of trying to explain the existence of ions is unnecessary as ions exist in nature, so to discuss ionic substances, one just needs to discuss the process of crystallisation from aqueous solution as a result of evaporation of the solvent.
• Finally, caution should be exercised in considering Lewis structures of ionic compounds (such as those exposed in the column on page 10 of the enriched text): one should be comfortable with the notion of Lewis structures of sodium ions, and Lewis structures of chloride ions, but Lewis structures of the compound NaCl may not make sense. Such structures fail to represent the extended structure of ionic compounds, and can only lend themselves to formation of images of single ion-pairs (or molecules).
Conflicts of interest
Acknowledgements.
- Atkins P., (1999), Chemistry: the great ideas, Pure Appl. Chem. , 71 (6), 927–929.
- Atkins P., (2010), Chemistry's core ideas, Chemistry Education in New Zealand , 2010, 8–12.
- Atkins P., (2014), Physical chemistry, a very short introduction , Oxford, UK: Oxford University Press.
- Ballester Pérez J. R., Ballester Pérez M. E., Calatayud M. L., García-Lopera R., Sabater Montesinos J. V. and Trilles Gil E., (2017), Student's misconceptions on chemical bonding: a comparative study between high school and first year university students, Asian Journal of Education and e-Learning , 5 (1), Asian Online Journals (http://www.ajouronline.com).
- Barker V. and Millar R., (2000), Students' reasoning about basic chemical thermodynamics and chemical bonding: what changes occur during a context-based post-16 chemistry course? Int. J. Sci. Educ. , 22 (11), 1171–1200.
- Bergqvist A., Drechsler M., De Jong O. and Chang Rundgren S.-N. C., (2013), Representations of chemical bonding models in school textbooks – help or hindrance for understanding? Chem. Educ. Res. Pract. , 14 (4), 589–606.
- Biggs J. B., (1999), Teaching for quality learning at university , Open University Press.
- Biggs J. B. and Collins K. F., (1982), Evaluating the quality of learning. The SOLO Taxonomy , New York, Academic Press.
- Birk J. P. and Kurtz M. J., (1999), Effect of experience on retention and elimination of misconceptions about molecular structure and bonding, J. Chem. Educ. , 76 (1), 124.
- Boo H.-K. and Watson J. R., (2001), Progression in high school students’ (aged 16–18) conceptualizations about chemical reactions in solution, Sci. Educ. , 85 (5), 568–585.
- Britton B. K., Woodward A. and Binkley M. (ed.), (1993), Learning from textbooks theory and practice , Hillsdale, New Jersey Hove and London: Lawrence Erlbaum Associates.
- Burrows N. L. and Reid Mooring S., (2015), Using concept mapping to uncover students’ knowledge structures of chemical bonding concepts, Chem. Educ. Res. Pract. , 16 (1), 53–66.
- Butts B. and Smith R., (1987), HSC chemistry students' understanding of the structure and properties of molecular and ionic compounds, Res. Sci. Educ. , 17 (1), 192–201.
- Cheng M. M. W. and Oon P.-T., (2016), Understanding metallic bonding: structure, process and interaction by Rasch analysis, Int. J. Sci. Educ. , 38 (12), 1923–1944.
- Coll R. K. and Taylor N., (2001), Alternative conceptions of chemical bonding held by upper secondary and tertiary students, Res. Sci. Tech. Educ. , 19 (2), 171–191.
- Coll R. K. and Treagust D. F., (2001), Learners’ mental models of chemical bonding, Res. Sci. Educ. , 31 (3), 357–382.
- Coll R. K. and Treagust D. F., (2002), Exploring tertiary students’ understanding of covalent bonding, Res. Sci. Tech. Educ. , 20 (2), 241–267.
- Gilbert J. K., (2006), On the nature of “context” in chemical education, Int. J. Sci. Educ. , 28 (9), 957–976.
- Gillespie R. J., (1997), The great ideas of chemistry, J. Chem. Educ. , 74 (7), 862–864.
- Harrison A. G. and Treagust D. F., (2000), Learning about atoms, molecules, and chemical bonds: a case study of multiple model use in grade 11 chemistry, Sci. Educ. , 84 (3), 352–381.
- Hazzi S. and Dumon A., (2014), Conceptual integration of covalent bond models by Algerian students, Chem. Educ. Res. Pract. , 15 (4), 675–688.
- Holme T. and Murphy K., (2012), The ACS Exams Institute undergraduate chemistry anchoring concepts map I: General chemistry, J. Chem. Educ. , 89 (6), 721–723.
- Holme T., Luxford C. and Murphy K., (2015), Updating the general chemistry anchoring concepts map, J. Chem. Educ. , 92 (6), 1115–1116.
- Kronik L., Levy Nahum T., Mamlok-Naaman R. and Hofstein A., (2008), A “bottom-up” framework for teaching chemical bonding, J. Chem. Educ. , 85 (12), 1680–1685.
- Levy Nahum T., Mamlok-Naaman R., Hofstein A. and Krajcik J., (2007), Developing a new teaching approach for the chemical bonding concept aligned with current scientific and pedagogical knowledge, Sci. Educ. , 91 (4), 579–603.
- Levy Nahum T., Mamlok-Naaman R., Hofstein A. and Taber K., (2010), Teaching and learning the concept of chemical bonding, Stud. Sci. Educ. , 46 (2), 179–207.
- Levy Nahum T., Mamlok-Naaman R. and Hofstein A., (2013), Teaching and learning of the chemical bonding concept: problems and some pedagogical issues and recommendations, in Tsaparlis G. and Sevian H. (ed.), Concepts of matter in science education , Dordrecht, the Netherlands: Springer, pp. 373–390.
- Liodakis S., Gakis D., Theodoropoulos D., Theodoropoulos P. and Kallis A., (2012), Chemistry for the 10th-grade general education , (in Greek), Athens, Greece: Greek Ministry of Education and Religious Affairs/Institute of Educational Policy/Diophantos, Institute of Technology, Computers, and Publications, pp. 52–61 and 74–77, http://ebooks.edu.gr/modules/document/file.php/DSGL111/%CE%94%CE%B9%CE%B4%CE%B1%CE%BA%CF%84%CE%B9%CE%BA%CF%8C%20%CE%A0%CE%B1%CE%BA%CE%AD%CF%84%CE%BF/%CE%92%CE%B9%CE%B2%CE%BB%CE%AF%CE%BF%20%CE%9C%CE%B1%CE%B8%CE%B7%CF%84%CE%AE/22-0215-02_Chimeia_A-Lyk_BM.pdf.
- Luxford C. J. and Bretz S. L., (2014), Development of the bonding representations inventory to identify student misconceptions about covalent and ionic bonding representations, J. Chem. Educ. , 91 (3), 312–320.
- Macintosh H. G. and Morrison R. B., (1969), Objective testing , London: University of London Press.
- Niaz M., (2001), A rational reconstruction of the origin of the covalent bond and its implications for general chemistry textbooks, Int. J. Sci. Educ. , 23 (6), 623–641.
- Nicoll G., (2001), A report of undergraduates’ bonding misconceptions, Int. J. Sci. Educ. , 23 (7), 707–730.
- Nimmermark A., Öhrström L., Mårtensson J. and Davidowitz B., (2016), Teaching of chemical bonding: a study of Swedish and South African students’ conceptions of bonding, Chem. Educ. Res. Pract. , 17 (4), 985–1005.
- Othman J., Treagust D. F. and Chandrasegaran A. L., (2008), An investigation into the relationship between students’ conceptions of the particulate nature of matter and their understanding of chemical bonding, Int. J. Sci. Educ. , 30 (11), 1531–1550.
- Özmen H., (2004), Some student misconceptions in chemistry: a literature review of chemical bonding, J. Sci. Educ. Technol. , 13 (2), 147–159.
- Pappa E. T. and Tsaparlis G., (2011), Evaluation of questions in general chemistry textbooks according to the form of the questions and the Question–Answer Relationship (QAR): the case of intra- and intermolecular chemical bonding, Chem. Educ. Res. Pract. , 12 (2), 262–270.
- Pearson P. D. and Johnson D. D., (1978), Teaching reading comprehension , New York: Holt, Rinehart and Winston.
- Peterson R. F. and Treagust D. F., (1989), Grade-12 students’misconceptions of covalent bonding and structure, J. Chem. Educ. , 66 (6), 459.
- Peterson R., Treagust D. F. and Garnett P., (1986), Identification of secondary students’ misconceptions of covalent bonding and structure concepts using a diagnostic instrument, Res. Sci. Educ. , 16 (1), 40–48.
- Peterson R. F., Treagust D. F. and Garnett P., (1989), Development and application of a diagnostic instrument to evaluate grade-11 and -12 students’ concepts of covalent bonding and structure following a course of instruction, J. Res. Sci. Teach. , 26 (4), 301–314.
- Robinson W. R., (1998), An alternative framework for chemical bonding, J. Chem. Educ. , 75 (9), 1074–1075.
- Schneider W., (2004), Metacognitive development: educational implications, in International Encyclopedia of the Social & Behavioral Sciences , pp. 9730–9733.
- Spiegel M. R., (1961), Theory and problems of statistics (Schaum's Ourline Series), New York: Mc-Graw-Hill.
- Taber K. S., (1994), Misunderstanding the ionic bond, Educ. Chem. , 31 (4), 100–103.
- Taber K. S., (1997), Student understanding of ionic bonding: molecular versus electrostatic thinking? Sch. Sci. Rev. , 78 (285), 85–95.
- Taber K. S., (1998), An alternative conceptual framework from chemistry education, Int. J. Sci. Educ. , 20 (5), 597–608.
- Taber K. S., (1999), Alternative conceptual frameworks in chemistry, Educ. Chem. , 36 (5), 135–137.
- Taber K. S., (2002a), Chemical misconceptions – Prevention, diagnosis and cure: Theoretical background , London: Royal Society of Chemistry, vol. 1.
- Taber K. S., (2002b), Chemical misconceptions – Prevention, diagnosis and cure: Classroom resources , London: Royal Society of Chemistry, vol. 2.
- Taber K. S., (2013), A common core to chemical conceptions: learners’ conceptions of chemical stability, change and bonding, in Tsaparlis G. and Sevian H. (ed.), Concepts of matter in science education , Dordrecht, The Netherlands: Springer, pp. 391–418.
- Taber K. S. and Coll R., (2003), Bonding, in Gilbert J. K., Jong O. D., Justy R., Treagust D. F. and Van Driel J. H. (ed.), Chemical education: towards research-based practice , Dordrect: Kluwer, pp. 213–234.
- Taber K. S., Tsaparlis G. and Nakiboĝlu C., (2012), Student conceptions of ionic bonding: patterns of thinking across three European contexts, Int. J. Sci. Educ. , 34 (18), 2843–2873.
- Talanquer V., (2007), Explanations and teleology in chemistry education, Int. J. Sci. Educ. , 29 (7), 853–870.
- Talanquer V., (2013), How do students reason about chemical substances and reactions? in Concepts of matter in science education , Tsaparlis G. and Sevian H. (ed.), Dordrechet, The Netherlands: Springer, pp. 331–346.
- Tan K.-C. D. and Treagust D. F., (1999), Evaluating students’ understanding of chemical bonding, Sch. Sci. Rev. , 81 (294), 75–83.
- Tsaparlis G. and Pappa E. T., (2012), Types of intra- and inter-molecular bonding: the case of general chemistry textbooks, in Bruguière C., Tiberghien A. and Clément P. (ed.), Proceedings of the ESERA 2011 Conference , Lyon, France, http://www.esera.org/publications/esera-conference-proceedings/.
- Ünal S., Çalik M., Ayas A. and Coll R., (2006), A review of chemical bonding studies: needs, aims, methods of exploring students’ conceptions, general knowledge claims and students’ alternative conceptions, Res. Sci. Tech. Educ. , 24 (2), 141–172.
- Ünal S., Coştu B. and Ayas A., (2010), Secondary school students’ misconceptions of covalent bonding, J. Turk. Sci. Educ. , 7 (2), 3–29.
- Vladušić R., Bucat R. B. and Ožić M., (2016), Understanding ionic bonding – a scan across the Croatian education system, Chem. Educ. Res. Pract. 17 (4), 685–699.
- Vrabec M. and Prokŝa M., (2016), Identifying misconceptions related to chemical bonding concepts in the Slovak school system using the bonding representations inventory as a diagnostic tool, J. Chem. Educ. , 93 (8), 1364–1370.
- Wang C.-Y. and Barrow L. C. R., (2013), Exploring conceptual frameworks of models of atomic structures and periodic variations, chemical bonding, and molecular shape and polarity: a comparison of undergraduate general chemistry students with high and low levels of content knowledge, Chem. Educ. Res. Pract. , 14 (1), 130–146.
- Yayon M., Mamlok-Naaman R. and Fortus D., (2012), Characterizing and representing students’ conceptual knowledge of chemical bonding, Chem. Educ. Res. Pract. , 13 (3), 248–267.

Gurukul of Excellence
Classes for Physics, Chemistry and Mathematics by IITians
Join our Telegram Channel for Free PDF Download
Case Study Questions for Class 11 Chemistry Chapter 4 Chemical Bonding and Molecular Structure
- Last modified on: 7 months ago
- Reading Time: 4 Minutes

Table of Contents
Case Study/Passage Based Questions:
Question 1:
Lewis dot structure of HCN molecule is given below. Answer questions on the basis of this structure.

(i) How many electrons are involved in the sigma bond between the carbon atom and the nitrogen atom? (A) 1 (B) 0 (C) 2 (D) 6
(ii) What types of bonds exist between the C atom and the N atom in HCN? (A) 3 sigma bonds and zero pi bonds (B) 3 pi bonds and zero sigma bonds (C) 2 pi bonds and one sigma bond (D) 1 pi bond and two sigma bonds
(iii) How many electrons does the N atom contribute to the pi bonds? (A) 3 (B) 2 (C) 1 (D) 5
Related Posts
Class 11 chemistry case study questions.
Chapter 1 Some Basic Concepts of Chemistry Case Study Questions Chapter 2 Structure of The Atom Case Study Questions Chapter 3 Classification of Elements and Periodicity in Properties Case Study Questions Chapter 4 Chemical Bonding and Molecular Structure Case Study Questions Chapter 5 States of Matter Case Study Questions Chapter 6 Thermodynamics Case Study Questions Chapter 7 Equilibrium Case Study Questions Chapter 8 Redox Reactions Case Study Questions Chapter 9 Hydrogen Case Study Questions Chapter 10 The s-Block Elements Case Study Questions Chapter 11 The p-Block Elements Case Study Questions Chapter 12 Organic Chemistry: Some Basic Principles and Techniques Case Study Questions Chapter 13 Hydrocarbons Case Study Questions Chapter 14 Environmental Chemistry Case Study Questions
Download CBSE Books
Exam Special Series:
- Sample Question Paper for CBSE Class 10 Science (for 2024)
- Sample Question Paper for CBSE Class 10 Maths (for 2024)
- CBSE Most Repeated Questions for Class 10 Science Board Exams
- CBSE Important Diagram Based Questions Class 10 Physics Board Exams
- CBSE Important Numericals Class 10 Physics Board Exams
- CBSE Practical Based Questions for Class 10 Science Board Exams
- CBSE Important “Differentiate Between” Based Questions Class 10 Social Science
- Sample Question Papers for CBSE Class 12 Physics (for 2024)
- Sample Question Papers for CBSE Class 12 Chemistry (for 2024)
- Sample Question Papers for CBSE Class 12 Maths (for 2024)
- Sample Question Papers for CBSE Class 12 Biology (for 2024)
- CBSE Important Diagrams & Graphs Asked in Board Exams Class 12 Physics
- Master Organic Conversions CBSE Class 12 Chemistry Board Exams
- CBSE Important Numericals Class 12 Physics Board Exams
- CBSE Important Definitions Class 12 Physics Board Exams
- CBSE Important Laws & Principles Class 12 Physics Board Exams
- 10 Years CBSE Class 12 Chemistry Previous Year-Wise Solved Papers (2023-2024)
- 10 Years CBSE Class 12 Physics Previous Year-Wise Solved Papers (2023-2024)
- 10 Years CBSE Class 12 Maths Previous Year-Wise Solved Papers (2023-2024)
- 10 Years CBSE Class 12 Biology Previous Year-Wise Solved Papers (2023-2024)
- ICSE Important Numericals Class 10 Physics BOARD Exams (215 Numericals)
- ICSE Important Figure Based Questions Class 10 Physics BOARD Exams (230 Questions)
- ICSE Mole Concept and Stoichiometry Numericals Class 10 Chemistry (65 Numericals)
- ICSE Reasoning Based Questions Class 10 Chemistry BOARD Exams (150 Qs)
- ICSE Important Functions and Locations Based Questions Class 10 Biology
- ICSE Reasoning Based Questions Class 10 Biology BOARD Exams (100 Qs)
✨ Join our Online JEE Test Series for 499/- Only (Web + App) for 1 Year
✨ Join our Online NEET Test Series for 499/- Only for 1 Year
Leave a Reply Cancel reply
Join our Online Test Series for CBSE, ICSE, JEE, NEET and Other Exams

Editable Study Materials for Your Institute - CBSE, ICSE, State Boards (Maharashtra & Karnataka), JEE, NEET, FOUNDATION, OLYMPIADS, PPTs
Discover more from Gurukul of Excellence
Subscribe now to keep reading and get access to the full archive.
Type your email…
Continue reading
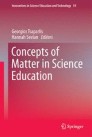
Concepts of Matter in Science Education pp 373–390 Cite as
Teaching and Learning of the Chemical Bonding Concept: Problems and Some Pedagogical Issues and Recommendations
- Tami Levy Nahum 3 ,
- Rachel Mamlok-Naaman 3 &
- Avi Hofstein 3
- First Online: 01 January 2013
2178 Accesses
7 Citations
Part of the book series: Innovations in Science Education and Technology ((ISET,volume 19))
This research consists of a diagnostic study, followed by a curricular development and implementation regarding the teaching of the bonding concept. The diagnostic study focuses on students’ difficulties over two decades as regards this concept. We focused on how the structure and content of the 12th-grade matriculation examinations conducted in Israel influence the way bonding is taught and assessed. The traditional pedagogical approach for teaching bonding is often overly simplistic and not aligned with the most up-to-date scientific ideas. Based on the analysis of this study and supported by researches worldwide, we suggest that the general approach of the bonding curriculum along with the current system of assessment causes students to study by rote memorization. We then describe the development of a new conceptual framework that provides an advanced scientific and pedagogical foundation for the teaching of bonding; this process was conducted with chemistry lead-teachers, senior chemistry educators, and eminent chemists. In general, we suggest adopting a new approach that rationalizes all bonds and structures based on a small set of underlying assumptions. We also suggest that one of the key goals of the proposed framework is to stress that a continuum scale exists between extreme cases of qualitatively different bonding scenarios. The research includes the implementation of this approach in ten classes, and our study indicates that both students and teachers acquired a much deeper understanding of the underling key concepts.
This is a preview of subscription content, log in via an institution .
Buying options
- Available as PDF
- Read on any device
- Instant download
- Own it forever
- Available as EPUB and PDF
- Compact, lightweight edition
- Dispatched in 3 to 5 business days
- Free shipping worldwide - see info
- Durable hardcover edition
Tax calculation will be finalised at checkout
Purchases are for personal use only
Ashkenazi, G., & Kosloff, R. (2006). The uncertainty principle and covalent bonding. The Chemical Educator, 11 , 66–76.
Google Scholar
Atzmon, A. (1991). Natural science curriculum as designer of the image of science using rhetorical patterns within the socio-political system . Unpublished doctoral dissertation, Hebrew University, Jerusalem (in Hebrew).
Ben-Zvi, R., Eylon, B., & Silberstein, J. (1986). Is an atom of copper malleable? Journal of Chemical Education, 63 , 64–66.
Article Google Scholar
Birenboum, M. (1997). Alternatives in assessment . Tel-Aviv: Ramot (in Hebrew).
Bodner, G., & Domin, D. (1998). Mental models: The role of representations in problem solving in chemistry . In International Council for Association in Science Education, Summer Symposium, Proceedings, Hong Kong.
Dori, Y. J. (2003). From nationwide standardized testing to school-based alternative embedded assessment in Israel: Students’ performance in the matriculation 2000 project. Journal of Research in Science Teaching, 40 , 34–52.
Erduran, S. (2003). Examining the mismatch between pupil and teacher knowledge in acid-base chemistry. School Science Review, 84 (308), 81–87.
Fensham, P. (1975). Concept formation. In D. J. Daniels (Ed.), New movements in the study and teaching of chemistry (pp. 199–217). London: Temple Smith.
Gabel, D. (1996, July). The complexity of chemistry: Research for teaching in the 21st century . Paper presented at the 14th International Conference on Chemical Education, Brisbane, Australia.
Gilbert, J. K. (2004). Models and modeling: Routes to more authentic science education. International Journal of Science and Mathematics Education, 2 , 115–130. doi: 10.1007/s10763-004-3186-4 .
Gilbert, J. K., Osborne, R. J., & Fensham, P. J. (1982). Children’s science and its consequences for teaching. Science Education, 66 , 623–633.
Gillespie, R. (1997). The great ideas of chemistry. Journal of Chemical Education, 74 , 862–864.
Glazer, N., Ben-Zvi, R., & Hofstein, A. (1999, March). The gap between factual knowledge and conceptual understanding in learning the concept “chemical bonding” . Paper presented at the annual meeting of the National Association for Research in Science Teaching, Boston.
Griffiths, A., & Preston, K. (1992). Grade-12 students’ misconceptions relating to fundamental characteristics of atoms and molecules. Journal of Research in Science Teaching, 29 , 611–628.
Harrison, A. G., & Treagust, D. F. (1996). Secondary students’ mental models of atoms and molecules: Implications for teaching chemistry. Science Education, 80 (5), 509–534.
Harrison, A. G., & Treagust, D. F. (2000). Learning about atoms, molecules, and chemical bonds: A case study of multiple-model use in grade 11 chemistry. Science Education, 84 , 352–381.
Henderleiter, J., Smart, R., Anderson, J., & Elian, O. (2001). How do organic chemistry students understand and apply hydrogen bonding? Journal of Chemical Education, 78 , 1126–1130.
Hurst, O. (2002). How we teach molecular structure to freshmen. Journal of Chemical Education, 79 (6), 763–764.
Justi, R., & Gilbert, J. (2002). Models and modeling in chemical education. In J. K. Gilbert, O. D. Jong, R. Justy, D. F. Treagust, & J. H. Van Driel (Eds.), Chemical education: Towards research-based practice (pp. 47–68). Dordrecht: Kluwer.
Kind, V. (2009). Pedagogical content knowledge in science education: Perspectives and potential for progress. Studies in Science Education, 45 , 169–204.
Levy Nahum, T. (2007). Teaching the concept of chemical bonding in high-school: Developing and implementing a new framework based on the analysis of misleading systemic factors . Unpublished doctoral dissertation, Weizmann Institute of Science, Rehovot, Israel (In Hebrew).
Levy Nahum, T., Hofstein, A., Mamlok-Naaman, R., & Bar-Dov, Z. (2004). Can final examinations amplify students’ misconceptions in chemistry? Chemistry Education Research and Practice in Europe, 5 (3), 301–325.
Levy Nahum, T., Mamlok-Naaman, R., Hofstein, A., & Krajcik, J. (2007). Developing a new teaching approach for the chemical bonding concept aligned with current scientific and pedagogical knowledge. Science Education, 91 , 579–603.
Levy Nahum, T., Mamlok-Naaman, R., Hofstein, A., & Kronik, L. (2008). A “bottom-up” framework for teaching chemical bonding. Journal of Chemical Education, 85 , 1680–1685.
Levy Nahum, T., Mamlok-Naaman, R., Hofstein, A., & Taber, K. (2010). Teaching and learning the concept of chemical bonding. Studies in Science Education, 46 (2), 179–207. doi: 10.1080/03057267.2010.504548 .
Lythcott, J. (1990). Problem solving and requisite knowledge of chemistry. Journal of Chemical Education, 67 , 248–252.
Morgan, D. L. (1997). Focus group as qualitative research (2nd ed.). London: Sage.
Pauling, L. (1967). The nature of the chemical bond, and the structure of molecules and crystals: An introduction to modern structural chemistry (3rd ed.). Ithaca: Cornell University Press.
Pellegrino, J. W., Chudowsky, N., & Glaser, R. (Eds.). (2001). Knowing what students know: The science and design of educational assessment . Washington, DC: National Academy Press.
Perkins, D. (1998). What is understanding? In M. S. Wiske (Ed.), Teaching for understanding: Linking research with practice . San Francisco: Jossey-Bass.
Reiser, B., Krajcik, J., Moje, E., & Marx, R. (2003). Design strategies for developing science instructional materials . Paper presented at the annual meeting of the National Association of Research in Science Teaching, Philadelphia.
Robinson, W. (2003). Chemistry problem-solving: Symbol, macro, micro, and process aspects. Journal of Chemical Education, 80 , 978–982.
Salloum, S., & Abd-El-Khalick, F. (2004). Relationships between selective cognitive variables and students’ ability to solve chemistry problems. International Journal of Science Education, 26 (1), 63–84.
Stinner, A. (1995). Science textbooks: Their present role and future form. In S. M. Glynn & R. Duit (Eds.), Learning science in the schools (pp. 275–296). Mahwah: Lawrence Erlbaum Associates.
Sutton, C. (1996). Beliefs about science and beliefs about language. International Journal of Science Education, 18 , 1–18.
Taagepera, M., Arasasingham, R., Potter, F., Soroudi, A., & Lam, G. (2002). Following the development of the bonding concept using knowledge space theory. Journal of Chemical Education, 79 (6), 756–762.
Taber, K. S. (1995). Development of student understanding: A case study of stability and liability in cognitive structure. Research in Science and Technological Education, 13 , 89–99.
Taber, K. S. (1998). An alternative conceptual framework from chemistry education. International Journal of Science Education, 20 , 597–608.
Taber, K. S. (2001a). Building the structural concepts of chemistry: Some considerations from educational research. Chemistry Education Research and Practice, 2 , 123–158.
Taber, K. S. (2001b). Shifting sands: A case study of conceptual development as competition between alternative conceptions. International Journal of Science Education, 23 , 731–753.
Taber, K. S. (2002a). Chemical misconceptions—Prevention, diagnosis and cure: Theoretical background (Vol. 1). London: Royal Society of Chemistry.
Taber, K. S. (2002b). Conceptualizing quanta: Illuminating the ground state of student understanding of atomic orbitals. Chemistry Education Research and Practice, 3 , 145–158.
Taber, K. S., & Coll, R. (2002). Bonding. In J. K. Gilbert, O. D. Jong, R. Justy, D. F. Treagust, & J. H. Van Driel (Eds.), Chemical education: Towards research-based practice (pp. 213–234). Dordrecht: Kluwer.
Taber, K. S., & Watts, M. (2000). Learners’ explanations for chemical phenomena. Chemistry Education Research and Practice in Europe, 1 (3), 329–353.
Teichert, M., & Stacy, A. (2002). Promoting understanding of chemical bonding and spontaneity through student explanation and integration of ideas. Journal of Research in Science Teaching, 39 (6), 464–496.
Treagust, D. F., & Harrison, A. G. (2000). In search of explanatory frameworks: An analysis of Richard Feynman’s lecture ‘Atoms in motion’. International Journal of Science Education, 11 , 1157–1170.
Tsaparlis, G. (1997). Atomic and molecular structure in chemical education. Journal of Chemical Education, 74 , 922–925.
Vinner, S. (1997). The pseudo-conceptual and the pseudo-analytical thought processes in mathematics learning. Educational Studies in Mathematics, 34 , 97–129.
Yifrach, M. (1999). Definition of chemical literacy and assessment of its attainment in high school chemistry . Unpublished master’s thesis, Weizmann Institute of Science, Rehovot (in Hebrew).
Download references
Author information
Authors and affiliations.
Department of Science Teaching, The Weizmann Institute of Science, Rehovot, 76100, Israel
Tami Levy Nahum, Rachel Mamlok-Naaman & Avi Hofstein
You can also search for this author in PubMed Google Scholar
Corresponding author
Correspondence to Avi Hofstein .
Editor information
Editors and affiliations.
, Department of Chemistry, University of Ioannina, Greece, University of Ioannina, Ioannina, 451 10, Greece
Georgios Tsaparlis
, Department of Chemistry, University of Massachusetts, Morrissey Blvd 100, Boston, 02125, Massachusetts, USA
Hannah Sevian
Rights and permissions
Reprints and permissions
Copyright information
© 2013 Springer Science+Business Media Dordrecht
About this chapter
Cite this chapter.
Nahum, T.L., Mamlok-Naaman, R., Hofstein, A. (2013). Teaching and Learning of the Chemical Bonding Concept: Problems and Some Pedagogical Issues and Recommendations. In: Tsaparlis, G., Sevian, H. (eds) Concepts of Matter in Science Education. Innovations in Science Education and Technology, vol 19. Springer, Dordrecht. https://doi.org/10.1007/978-94-007-5914-5_18
Download citation
DOI : https://doi.org/10.1007/978-94-007-5914-5_18
Published : 15 May 2013
Publisher Name : Springer, Dordrecht
Print ISBN : 978-94-007-5913-8
Online ISBN : 978-94-007-5914-5
eBook Packages : Humanities, Social Sciences and Law Education (R0)
Share this chapter
Anyone you share the following link with will be able to read this content:
Sorry, a shareable link is not currently available for this article.
Provided by the Springer Nature SharedIt content-sharing initiative
- Publish with us
Policies and ethics
- Find a journal
- Track your research
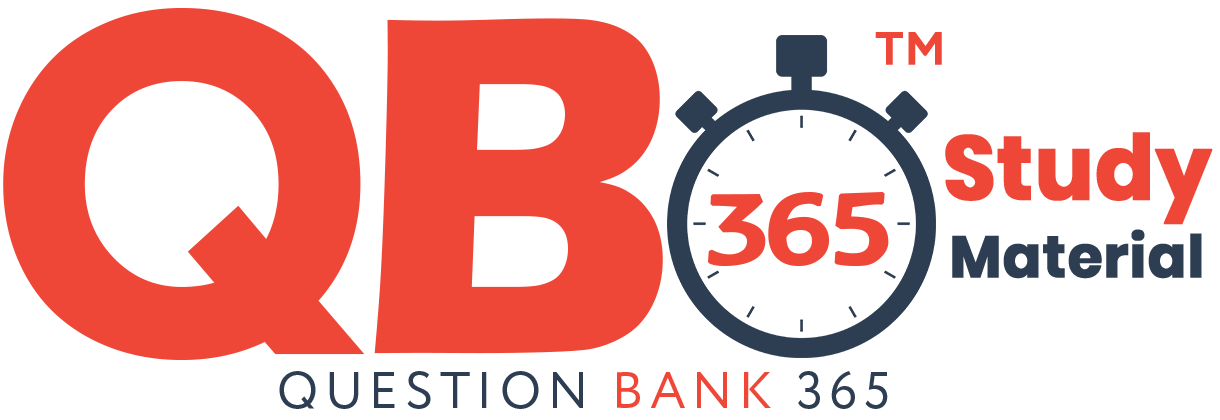
- Neet Online Test Pack
12th Standard stateboard question papers & Study material
தமிழ் subjects.
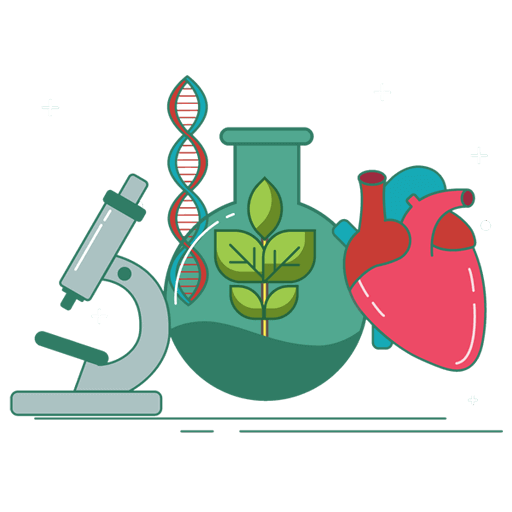
கணினி பயன்பாடுகள்
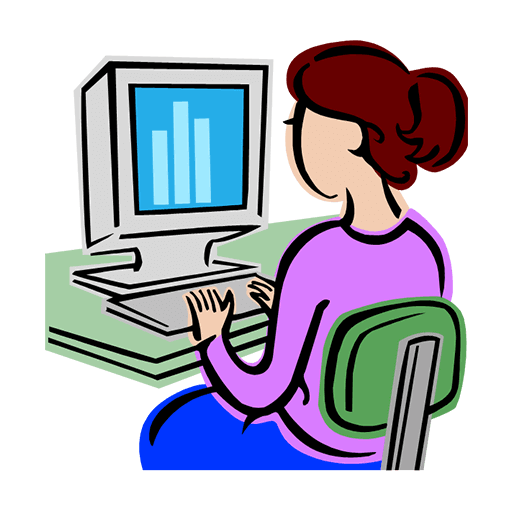
கணினி அறிவியல்
வணிகக் கணிதம் மற்றும் புள்ளியியல்.
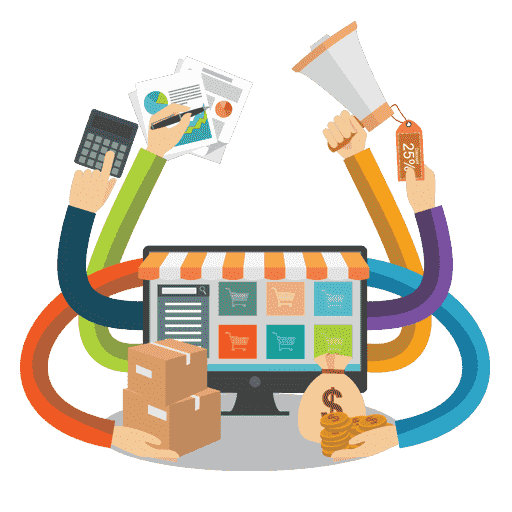
கணினி தொழில்நுட்பம்
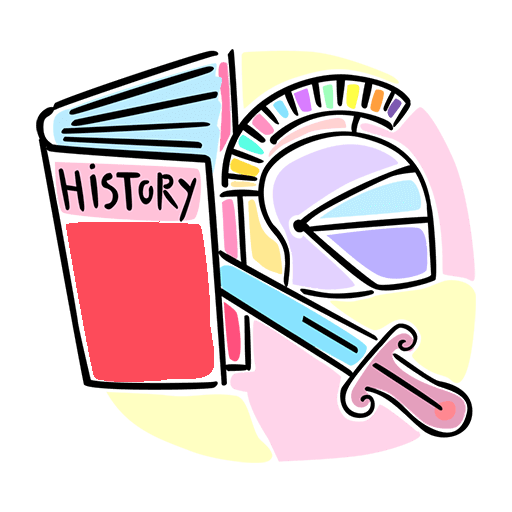
கணக்குப்பதிவியல்
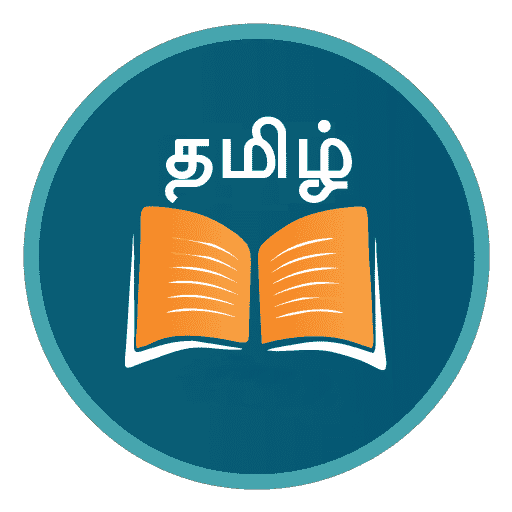
English Subjects
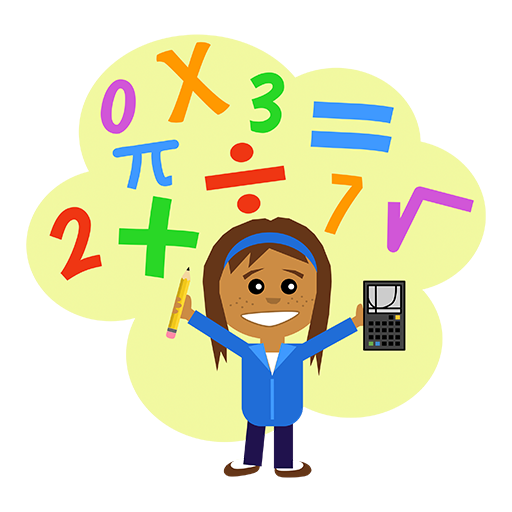
Computer Science
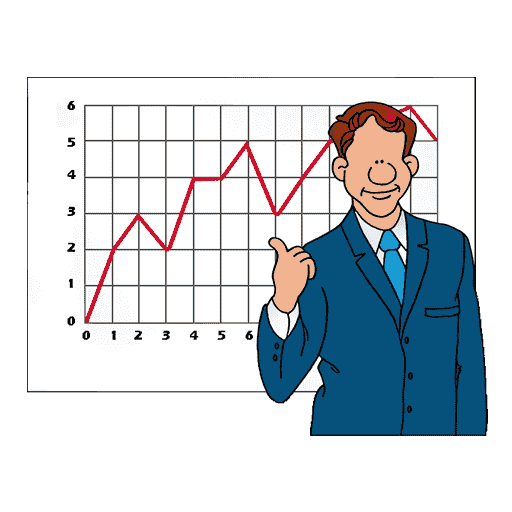
Business Maths and Statistics
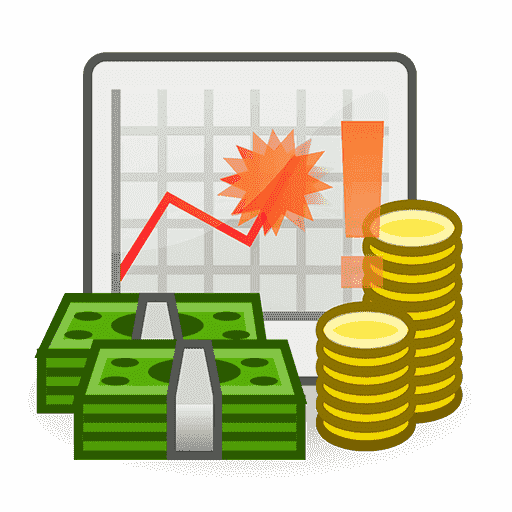
Accountancy
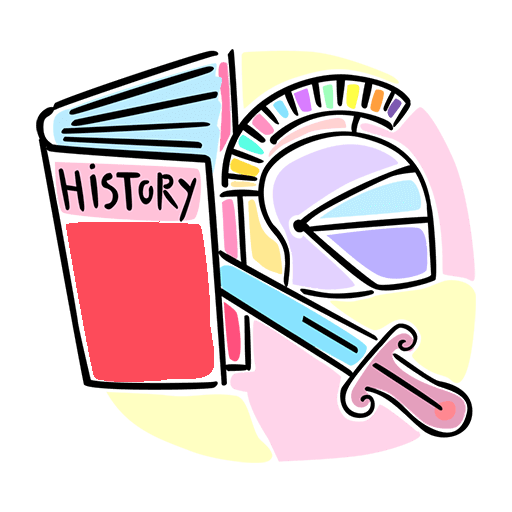
Computer Applications
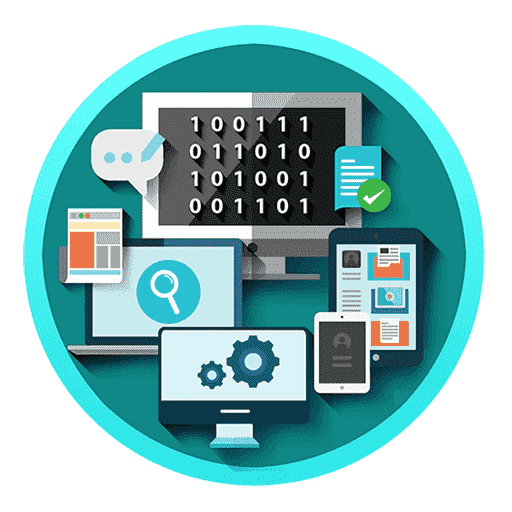
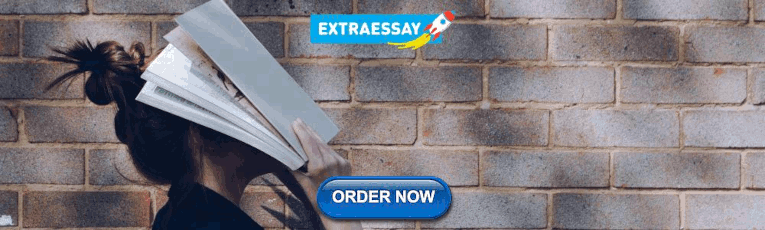
Computer Technology
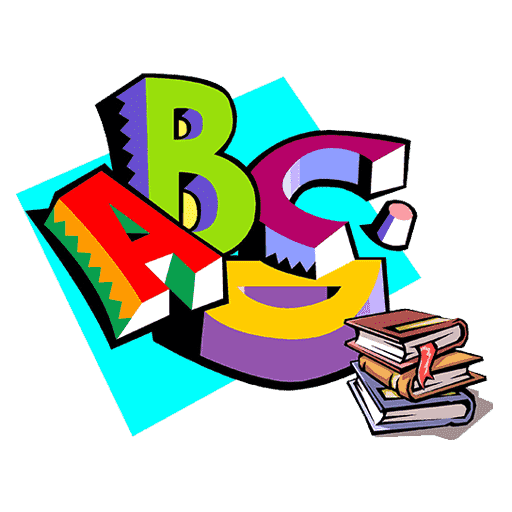
11th Standard stateboard question papers & Study material
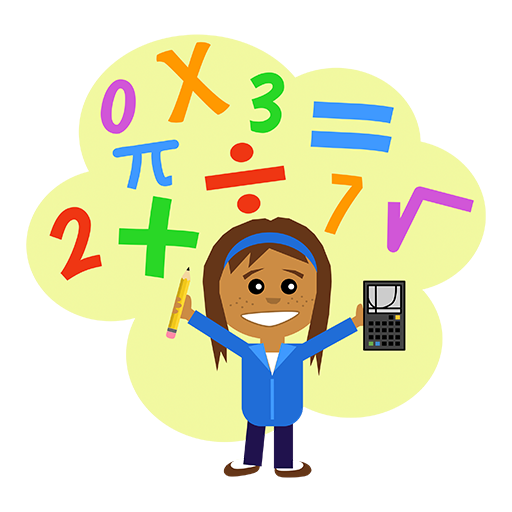
9th Standard stateboard question papers & Study material
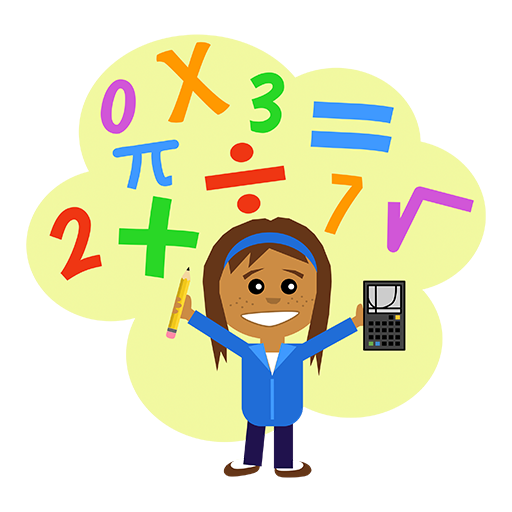
Social Science
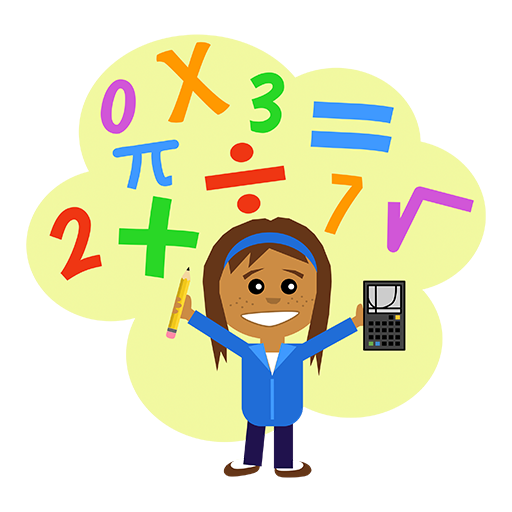
சமூக அறிவியல்
6th standard stateboard question papers & study material.
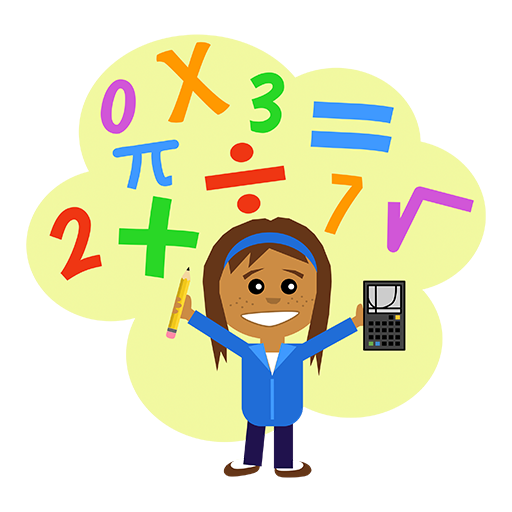
10th Standard stateboard question papers & Study material
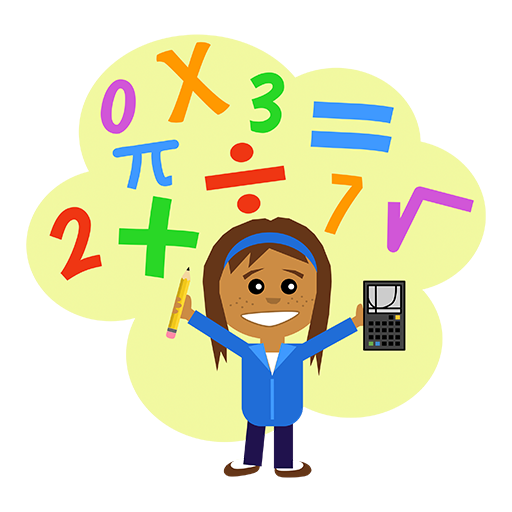
7th Standard stateboard question papers & Study material
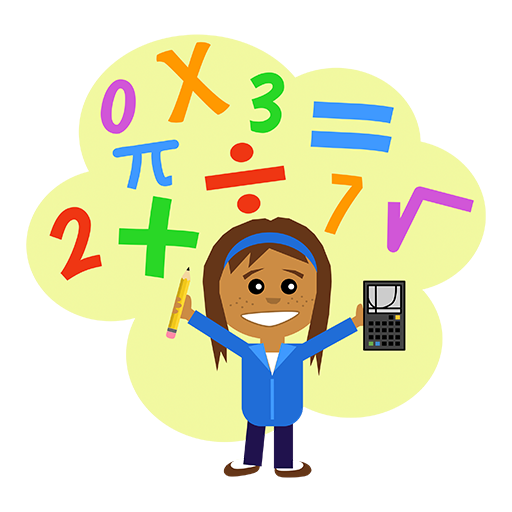
8th Standard stateboard question papers & Study material
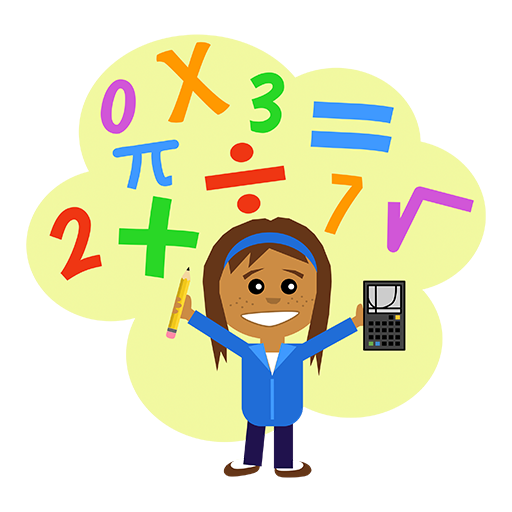
கணிதம் - old
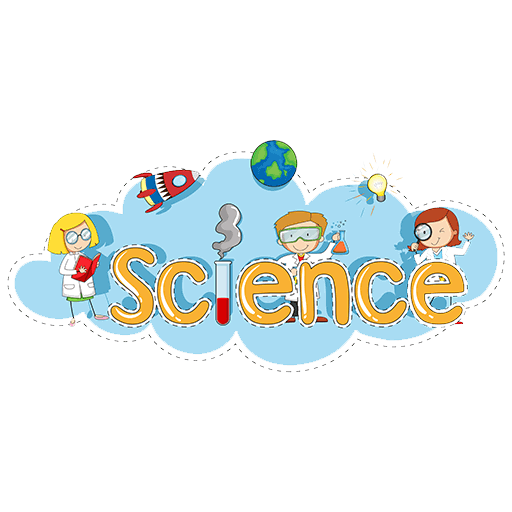
12th Standard CBSE Subject Question Paper & Study Material
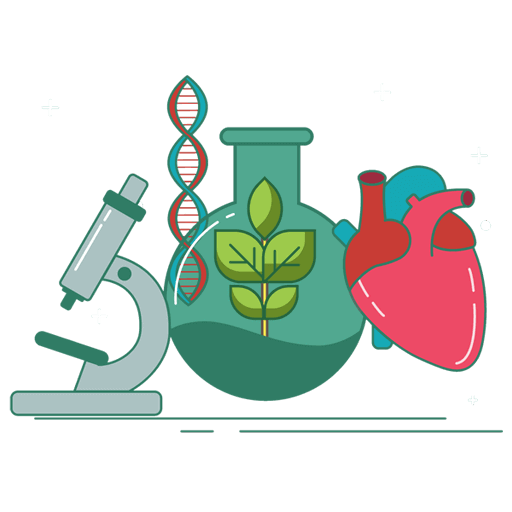
Introductory Micro and Macroeconomics
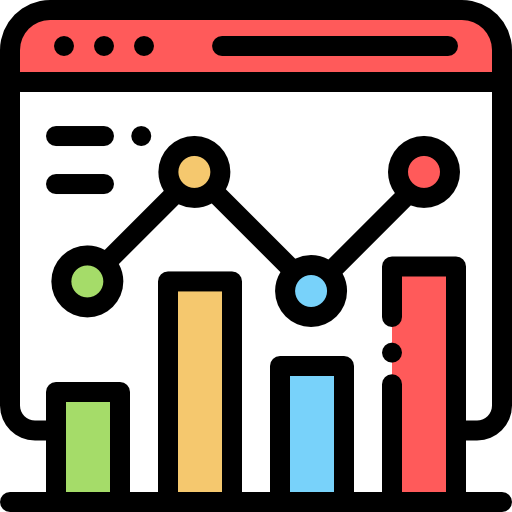
Business Studies
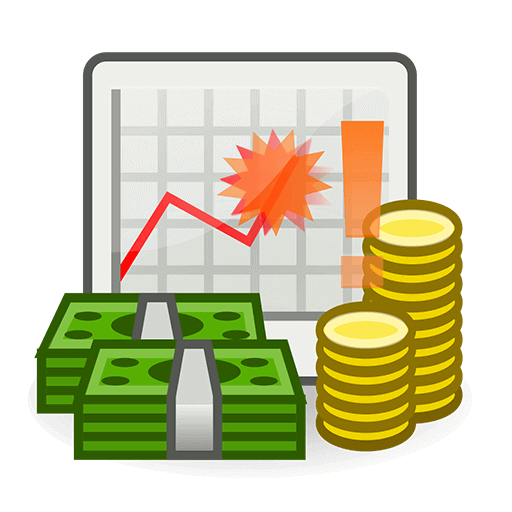
Indian Society

Physical Education
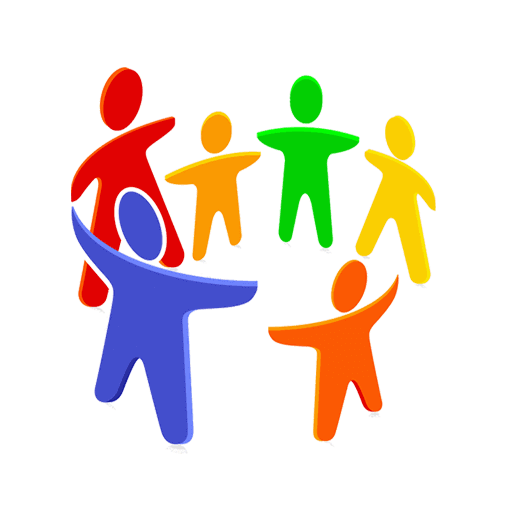
Bio Technology
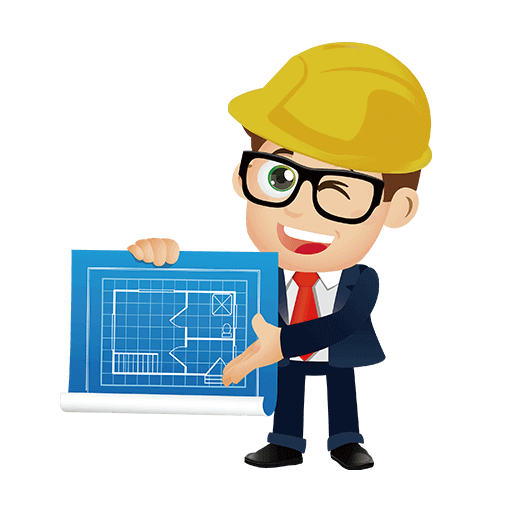
Engineering Graphics
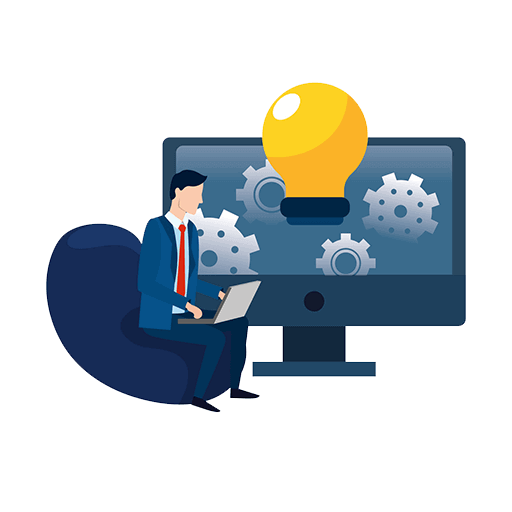
Entrepreneurship
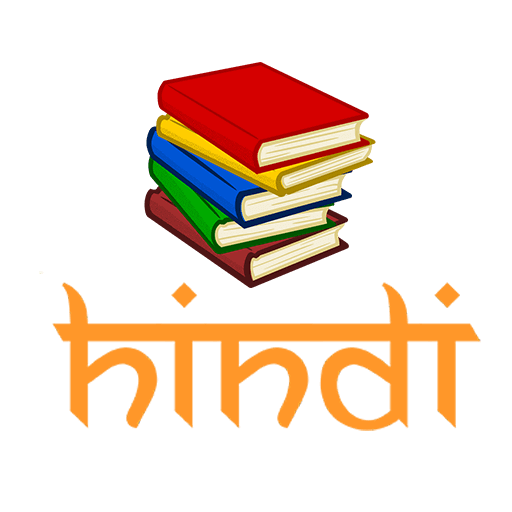
Hindi Elective
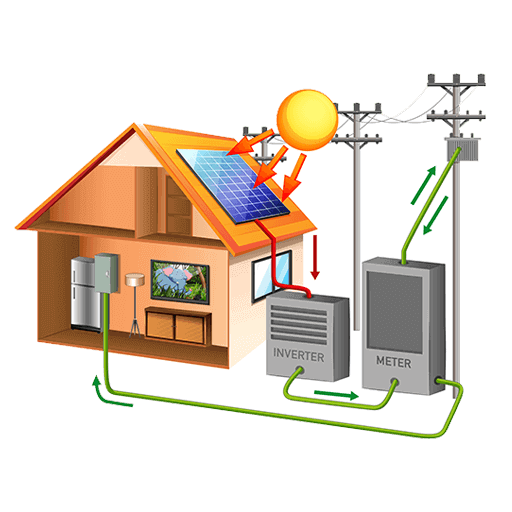
Home Science
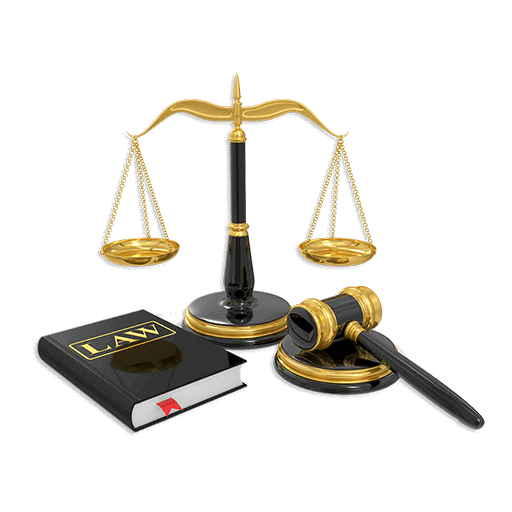
Legal Studies
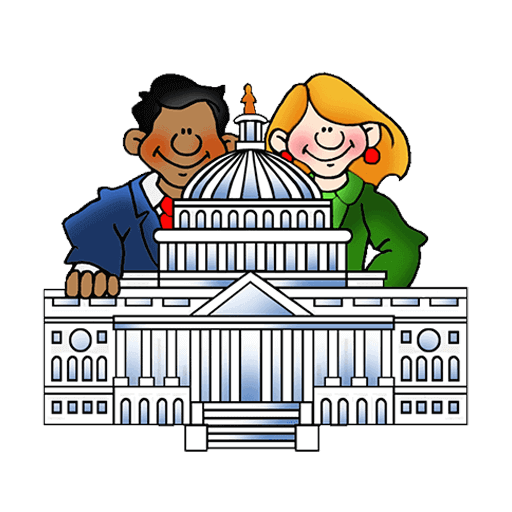
Political Science
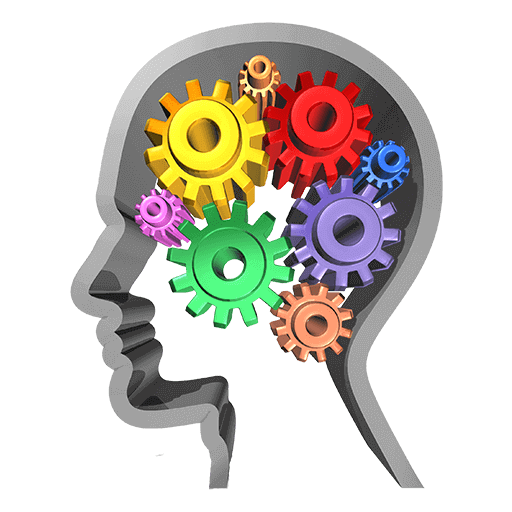
11th Standard CBSE Subject Question Paper & Study Material
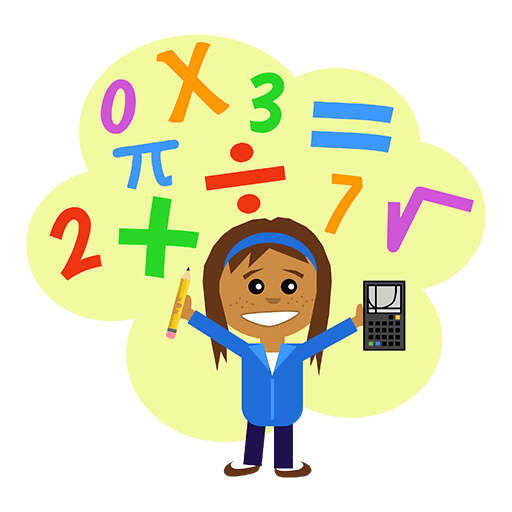
Mathematics
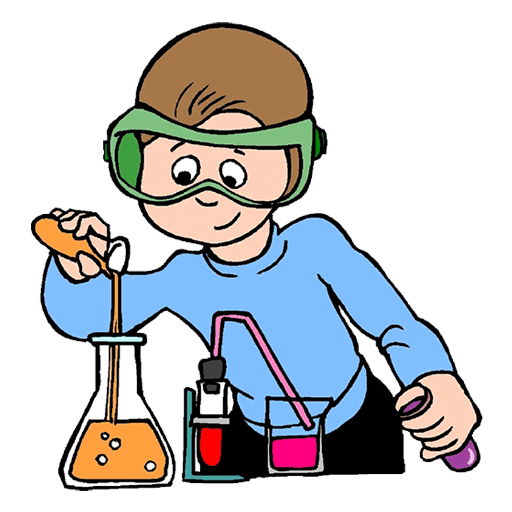
Enterprenership
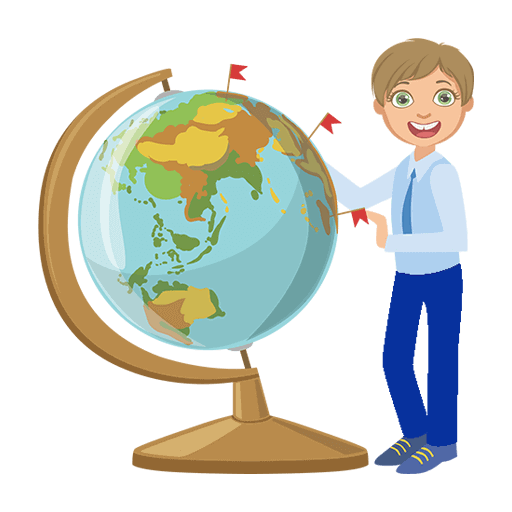
Applied Mathematics
10th standard cbse subject question paper & study material.
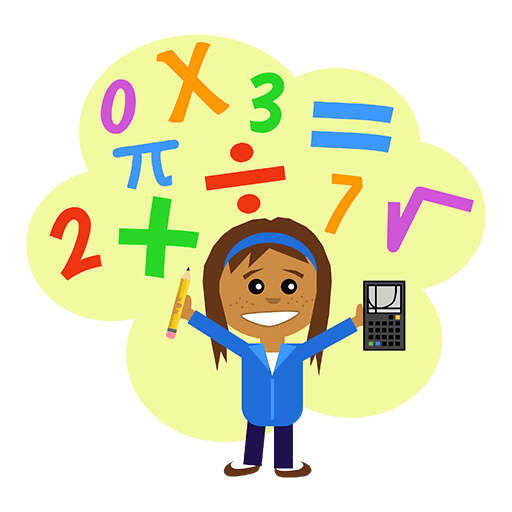
9th Standard CBSE Subject Question Paper & Study Material
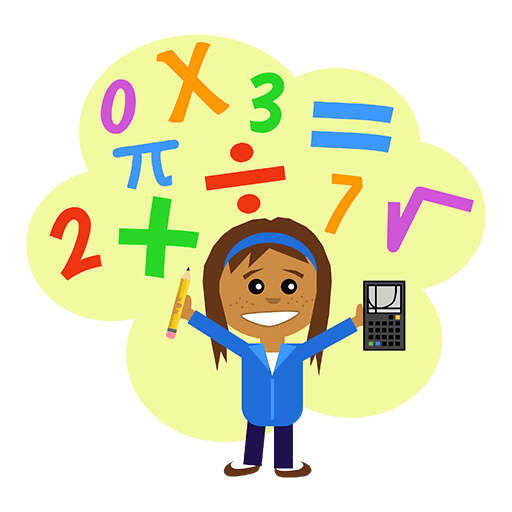
8th Standard CBSE Subject Question Paper & Study Material
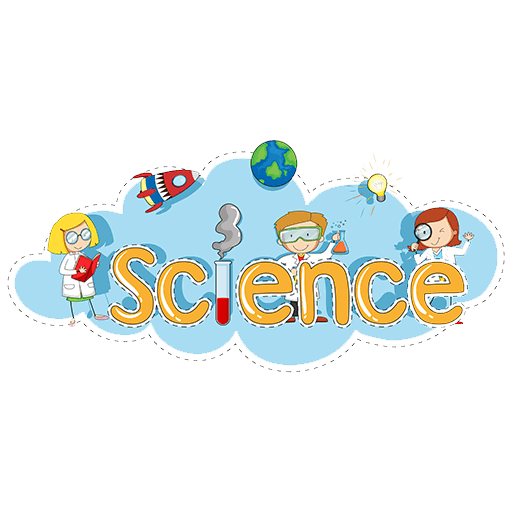
7th Standard CBSE Subject Question Paper & Study Material
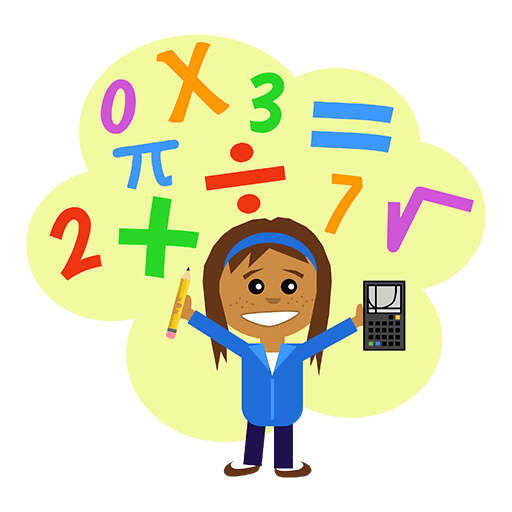
6th Standard CBSE Subject Question Paper & Study Material
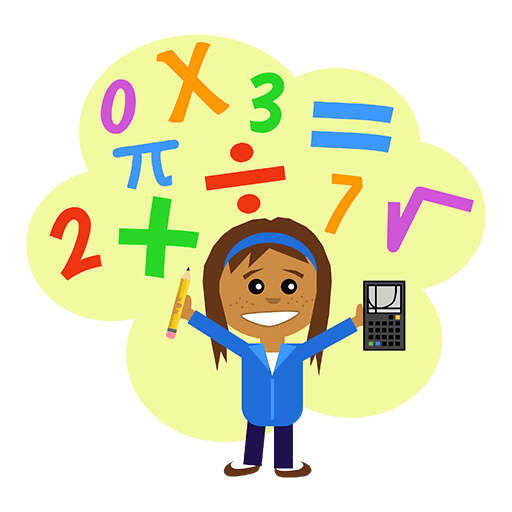
School Exams
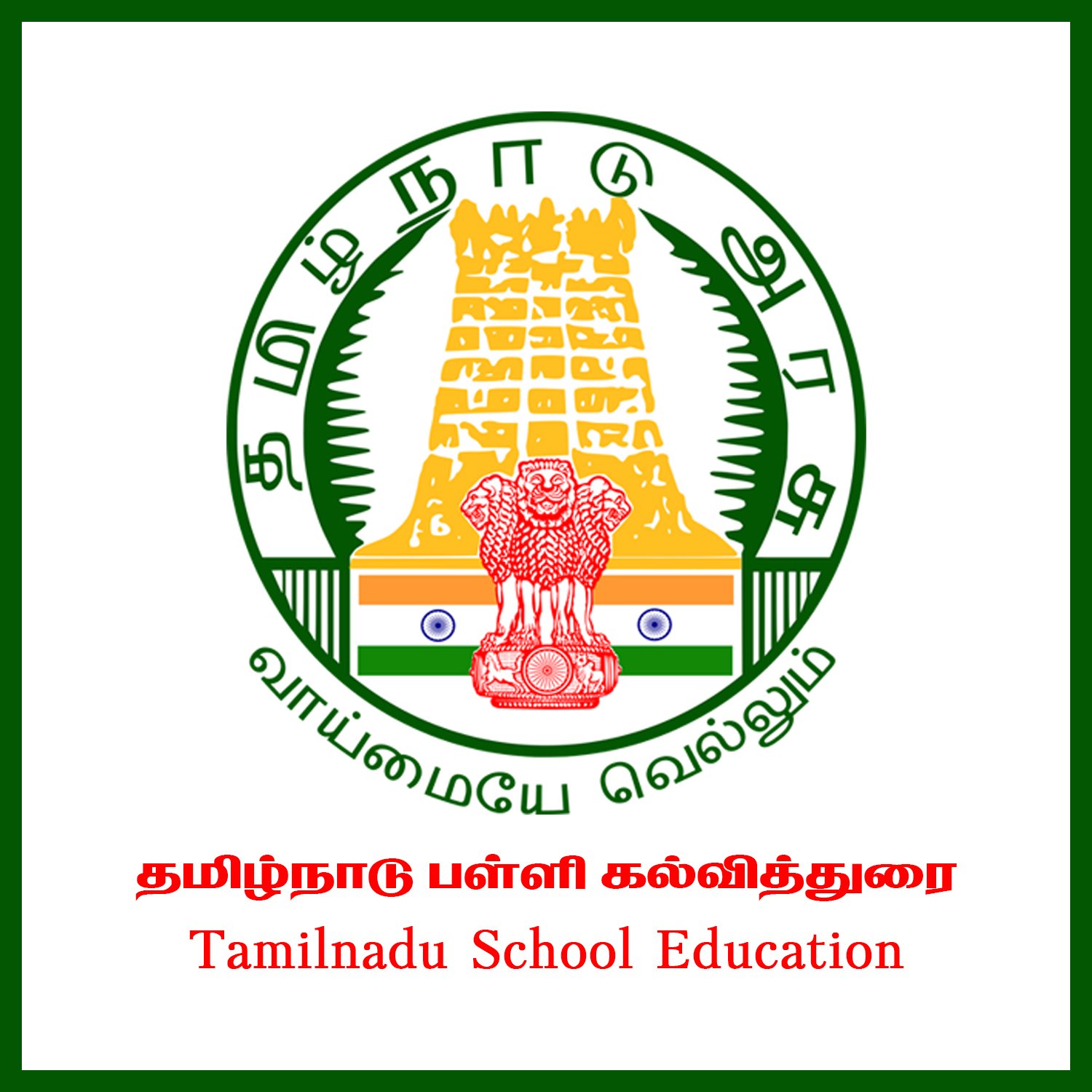
Tamil Nadu State Board Exams

Scholarship Exams
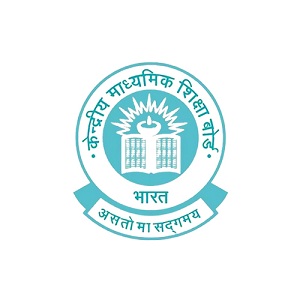
Study Materials , News and Scholarships
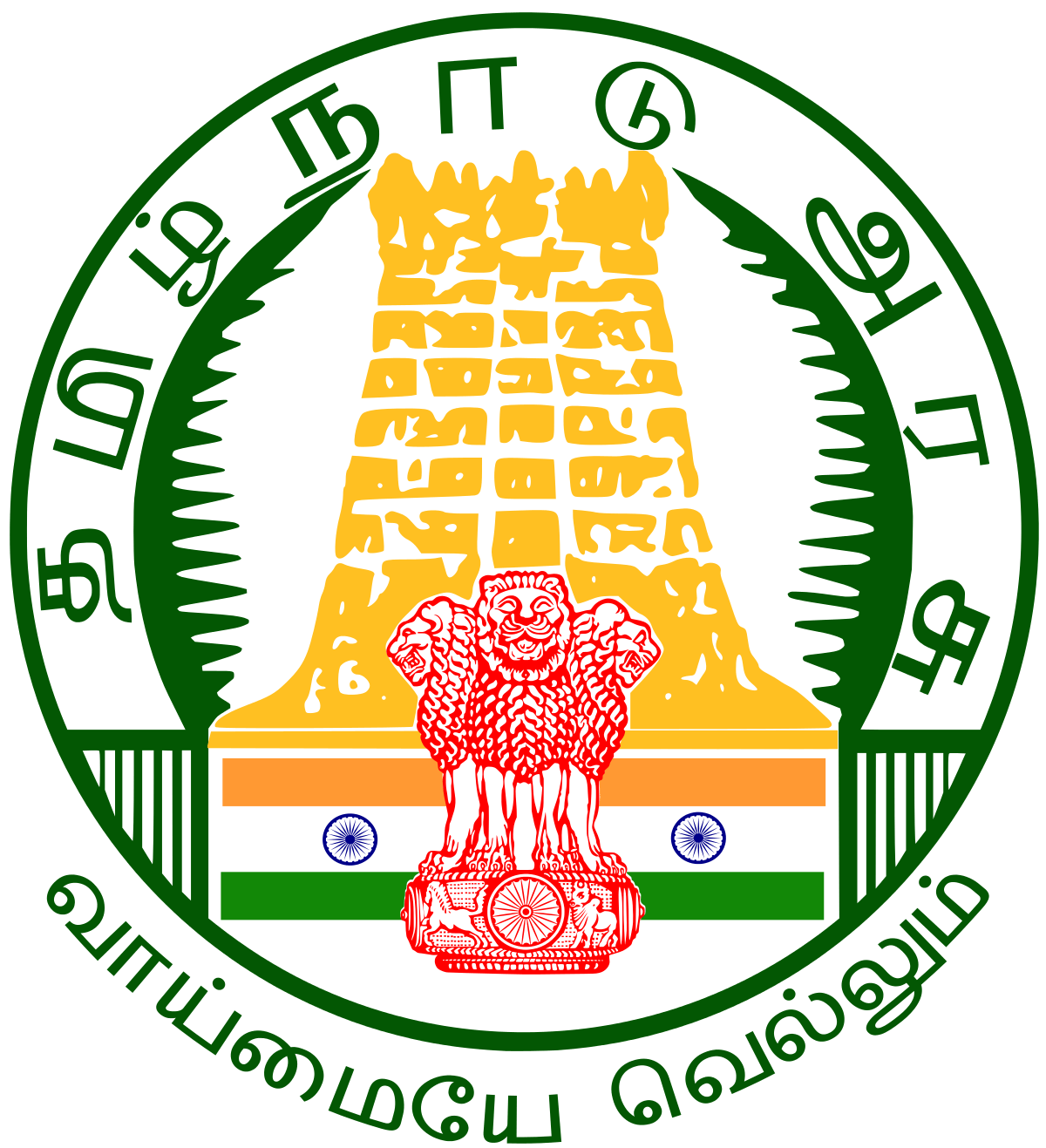
Stateboard Tamil Nadu
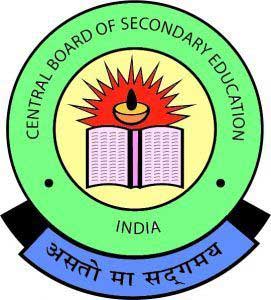
Free Online Tests

Educational News

Scholarships

Entrance Exams India
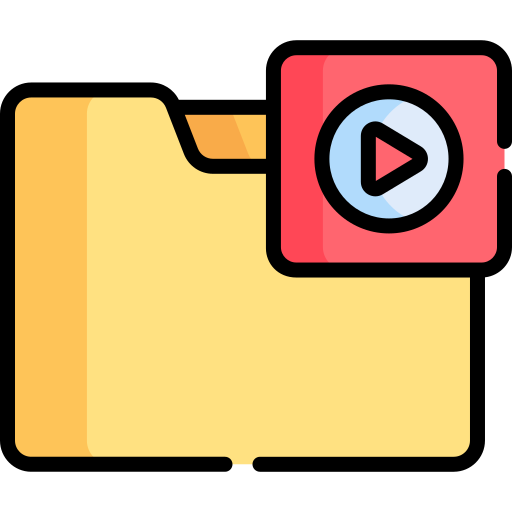
Video Materials

11th Standard CBSE
Class 11th Chemistry - Chemical Bonding and Molecular Structure Case Study Questions and Answers 2022 - 2023
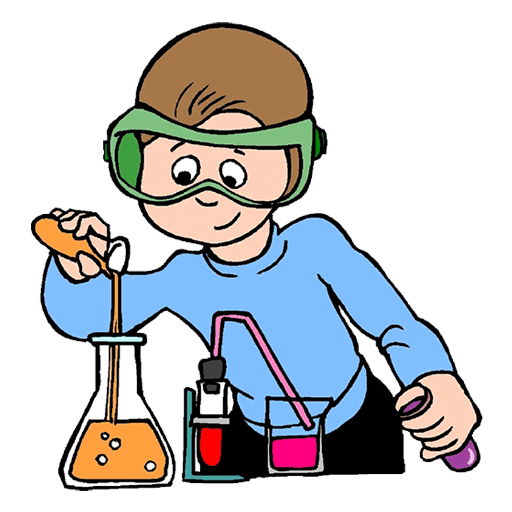
Class 11th Chemistry - Chemical Bonding and Molecular Structure Case Study Questions and Answers 2022 - 2023 Study Materials Sep-09 , 2022
QB365 provides a detailed and simple solution for every Possible Case Study Questions in Class 11 Chemistry Subject - Chemical Bonding and Molecular Structure, CBSE. It will help Students to get more practice questions, Students can Practice these question papers in addition to score best marks.

A PHP Error was encountered
Severity: Warning
Message: in_array() expects parameter 2 to be array, null given
Filename: material/details.php
Line Number: 1436
Message: Use of undefined constant EXAM - assumed 'EXAM' (this will throw an Error in a future version of PHP)
Line Number: 1438
QB365 - Question Bank Software
Chemical bonding and molecular structure case study questions with answer key.
Final Semester - June 2015
The attractive force which holds the two atoms together is called chemical bond. Covalent bond is formed by equal sharing of electrons. Coordinate bond is formed by unequal sharing of electrons. Ionic bond is formed by transfer of electrons from one atom to another. Octet rule, although very useful but it is not universally applicable. According to valence bond theory, covalent bond is formed by overlapping of half filled atomic orbitals resulting in lowering of energy and more stability. Bond order is the number of bonds between atoms in a molecule. Higher the bond order, more will be stability and bond dissociation enthalpy but smaller bond length. Polarity of covalent bond depends upon difference in electronegativity. Covalent character of bond depends upon polarising power, smaller cation and bigger anions have higher polarising power. VSEPR theory helps to predict shapes of molecules. (a) Write the, electron dot structure of N 2 O. (b) What are ions present in CsI 3 ? (c) Out of CN + , CN - , NO, which has highest bond order? (d) What is correct order of repulsion bp - bp, lp - lp and lp - bp? (e) Draw the structure of XeOF 4 on the basis of VSEPR theory. (f) Which out of B 2 ,CO, \(\mathrm{O}_{2}^{2-}\) and NO + are paramagnetic and why?
An ionic compound has 3-D crystal lattice in which positive and negative charges are equal. The crystal lattice is stabilised by enthalpy of lattice formation, bond length, bond angle, bond enthalpy, bond order and bond polarity have significant effect on properties of compounds. All the properties of certain compounds cannot be explained by single structure, more than one structure of a compound to explain its property are called resonating structures. Dipole moment depends upon polarity and shapes of molecules. Shapes of molecules can be determined by VSEPR theory as well as hybridisation sp, Sp 2 , Sp 3, Sp 3 d, Sp 3 d 2 are linear, trigonal planar, tetrahedral, trigonal bipyramidal and octahedral geometery respectively. Hydrogen bond is formed between hydrogen and F, O, N. Intra-molecular H-bonding is within the molecules which is weaker than inter molecular H-bonding, between the molecules. (a) Why does CO 2 have zero dipole moment? (b) What is hybridisation of 'S' in SF 6 and its shape? (c) Why do all bonds in \(\mathrm{CO}_{3}^{2-}\) h ave equal bond length? (d) Why is o-nitropbenol steam volatile, p-nitropbenol is not? (e) Wby is bond angle in H 2 O is more tban H 2 S? (f) Why is bond \(\sigma\) stronger than \(\pi\) -bond? (g) Arrange NaCl, NaBr, NaF. NaI in increasing order of ionic character.
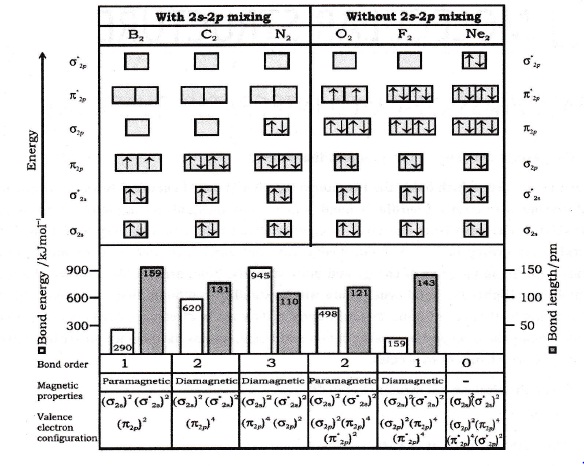
*****************************************
- Previous Class 11th Chemistry - Environmental Chemistry Case Study Questions and Answers ...
- Next Class 11th Chemistry - Hydrocarbons Case Study Questions and Answers 2022 - 2023
Reviews & Comments about Class 11th Chemistry - Chemical Bonding and Molecular Structure Case Study Questions and Answers 2022 - 2023
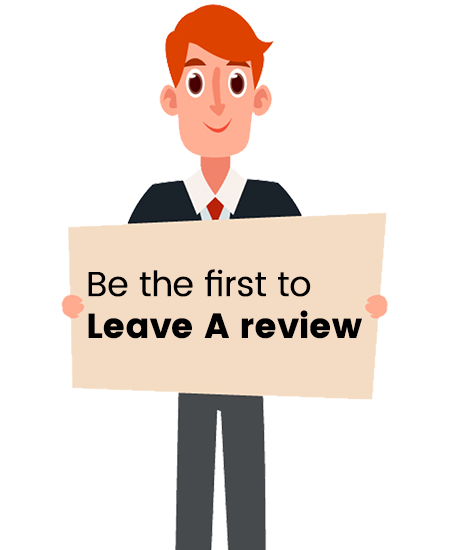
Write your Comment
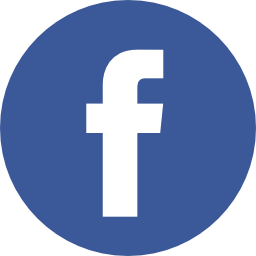
11th Standard CBSE Chemistry Videos
CBSE 11th Chemistry Sample Model Question Paper with Answer Key 2023
11th Standard CBSE Chemistry Usefull Links
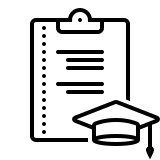
- 10th Standard
Other 11th Standard CBSE Subjects
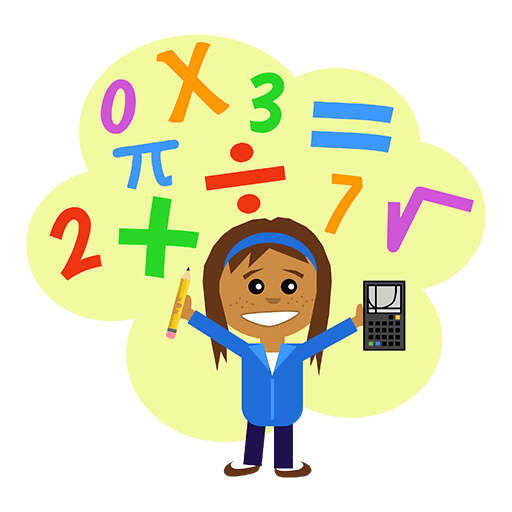
Other 11th Standard CBSE Chemistry Study material
Class 11th chemistry - environmental chemistry case ... click to view, class 11th chemistry - hydrocarbons case study questions and answers 2022 - 2023 click to view, class 11th chemistry - organic chemistry: some ... click to view, class 11th chemistry - the p-block elements ... click to view, class 11th chemistry - the s-block elements ... click to view, class 11th chemistry - hydrogen case study questions and answers 2022 - 2023 click to view, class 11th chemistry - redox reactions case ... click to view, class 11th chemistry - equilibrium case study questions and answers 2022 - 2023 click to view, class 11th chemistry - thermodynamics case study ... click to view, class 11th chemistry - state of matter ... click to view, class 11th chemistry - chemical bonding and ... click to view, class 11th chemistry - classification of elements ... click to view, class 11th chemistry - structure of atom ... click to view, class 11th chemistry - some basic concept ... click to view, 11th standard cbse mathematics public exam model question 2020 click to view, register & get the solution for class 11th chemistry - chemical bonding and molecular structure case study questions and answers 2022 - 2023.


- Book Solutions
- State Boards
ICSE Class 10 Chemistry Case Study based questions – Chemical Bonding
On this page we have given ICSE Class 10 Chemistry Chapter 2 Case Study based questions – Chemical Bonding. From 2024 Board exam, ICSE will focus on Technical Based, Analytical Based and Critical Questions in Chemistry Exam. So we Net Ex, has started giving Case Study based questions which is also known as Paragraph Based Questions.
Que No. 1 –
The molecule formed due to the sharing ofelectrons (covalent bond) is called a covalent molecule.The covalency of an atom is the number of itselectrons taking part in the formation of sharedpairs.The bond formed between two atoms (1) with same electronegativity is non-polar(2) with slightly different electronegativity ispolar.
1.) What are the different types of covalent bonds ?
2.) Define polar and non-polar covalent compounds ?
3.) What are the covalencies of hydrogen, oxygen, nitrogen and carbon ?
Que No. 2 –
The bond formed between two atoms by sharing a pair of electrons, provided entirely by one of the combining atoms but shared by both is called a co-ordinate bond.
1.) Write any two examples of the compounds formed by coordinate bonds
2.) Why coordinate bonds also called as dative bond or co-ionic bond ?
3.) What are Doner and Acceptor in coordinate compounds ?
Que No. 3 –
The cation and the anion being oppositely charged attract each other and form a chemical bond. Since this chemical bond formation is due to the electrostatic force of attraction between a cation and an anion, it is called an electrovalent (or an ionic bond).
1.) What are the Conditions for the formation of an electrovalent (or ionic bond)?
2.) What is metallic and non-metallic elements ?
3.) Which element from group 17 is the most electronegative in nature?
Que No. 1 Answers-
1.) Covalent bonds are of following three types:1)Single covalent bond 2) Double covalent bond 3)Triple covalent bond.
2.) The covalent compounds are said to be polarwhen the shared pair of electrons are not at equaldistance between the two atoms and Covalent compounds are non-polar when sharedpair of electron(s) are equally distributed betweenthe two atoms. No charge separation takes place.
3.) The covalency of hydrogen is 1,oxygen 2, nitrogen 3 and carbon 4.
Que No. 2 Answers-
1.) The two examples of coordinate bonds are 1) Ammonia 2)Water.
2.) A coordinate bond has properties of both covalent and ionic bonds. Therefore, it is also called dative or co-lonic bond.
3.) The atom which provides the electron pair for the formation of a coordinate bond is known as the DONOR and the atom or ion sharing the donated electron pair is known as the ACCEPTOR.
Que No. 3 Answers-
1.) The following three conditions are essential for the formation of electrovalent bond 1) Low ionization potential 2) High electron affinity 3)Large electronegativity difference.
2.) A non-metallic element, whose atom readily accepts electrons to form a negatively charged ion is an electronegative element and a metallic element, whose atom readily looses electrons to form a positively charged ion is an electropositive element.
3.) Fluorine is the element from group 17 is the most electronegative in nature.
Also See : This Chapter MCQ Question Answer
Leave a Reply Cancel reply
Your email address will not be published. Required fields are marked *
Save my name, email, and website in this browser for the next time I comment.
We have a strong team of experienced Teachers who are here to solve all your exam preparation doubts
Justify the statement “industrialisation and urbanisation go hand in hand” in details, creative hindi worksheet for lkg students in pdf, west bengal board class 9 bengali radharani solution, 10 anuched lekhan in hindi for class 10th students.
Sign in to your account
Username or Email Address
Remember Me
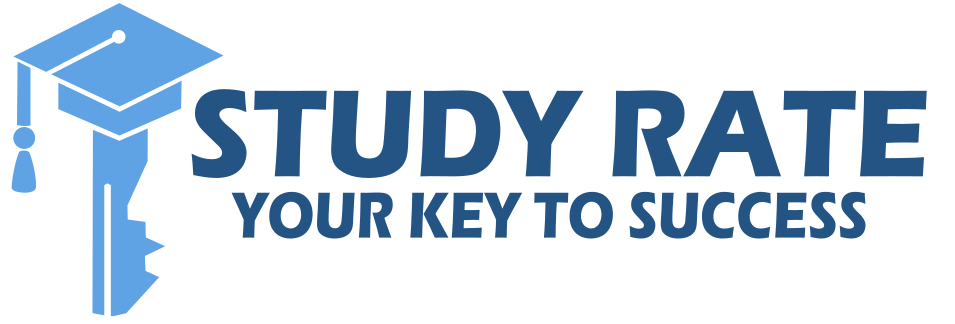
Class 11 Chemistry Case Study Questions Chapterwise PDF Download
- Post author: studyrate
- Post published:
- Post category: Chemistry / Class 11
- Post comments: 0 Comments
Case Study questions for the Class 11 Chemistry final exams are available here. For your Chemistry exam, you can read these chapter-by-chapter Case Study questions. Subject matter specialists and seasoned teachers created these quizzes. You can verify the right response to each question by referring to the answer key, which is also provided. To achieve success on your final exams, practice the following questions.
Join our Telegram Channel, there you will get various e-books for CBSE 2024 Boards exams for Class 9th, 10th, 11th, and 12th.
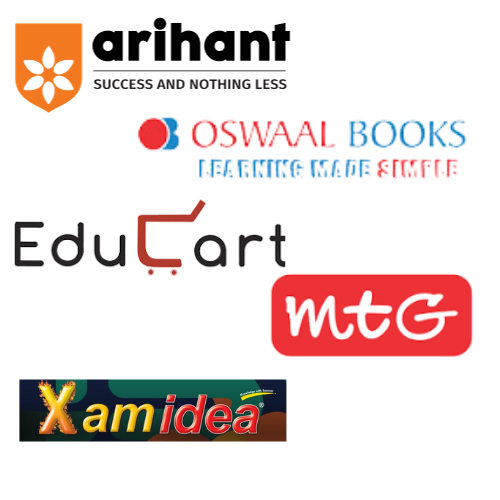
Class 11 Chemistry is divided into intriguing chapters, each unfolding a different aspect of chemical science. From understanding atomic structures to diving into organic chemistry, it’s a vast field.
In this article, we’ll delve into various aspects of Class 11 Chemistry Case Study Questions, exploring everything from the basics to complex analytical techniques. By the time you finish reading, you’ll have gained insights and valuable information that can enhance your understanding and performance.
Table of Contents
CBSE Class 11th Chemistry: Chapterwise Case Study Question & Solution
CBSE will ask two Case Study Questions in the CBSE class 11 Chemistry questions paper. Question numbers 15 and 16 are case-based questions where 5 MCQs will be asked based on a paragraph. Each theme will have five questions and students will have a choice to attempt any four of them.
Case Study-Based Questions for Class 11 Chemistry
- Case Study Based Questions on Class 11 Chemistry Chapter 1 Some Basic Concepts of Chemistry
- Case Study Based Questions on Class 11 Chemistry Chapter 2 Structure of Atom
- Case Study Based Questions on Class 11 Chemistry Chapter 3 Classification of Elements and Periodicity in Properties
- Case Study Based Questions on Class 11 Chemistry Chapter 4 Chemical Bonding and Molecular Structure
- Case Study Based Questions on Class 11 Chemistry Chapter 5 States of Matter
- Case Study Based Questions on Class 11 Chemistry Chapter 6 Thermodynamics
- Case Study Based Questions on Class 11 Chemistry Chapter 7 Equilibrium
- Case Study Based Questions on Class 11 Chemistry Chapter 8 Redox Reactions
- Case Study Based Questions on Class 11 Chemistry Chapter 10 The s-block elements
- Case Study Based Questions on Class 11 Chemistry Chapter 11 The p-block elements
- Case Study Based Questions on Class 11 Chemistry Chapter 12 Organic Chemistry -Some Basic Principles and Techniques
- Case Study Based Questions on Class 11 Chemistry Chapter 13 Hydrocarbons
Class 11 Chemistry M CQ Questions
Before the exams, students in class 11 should review crucial Chemistry Case Study issues. They will gain a better understanding of the kinds of Case Study questions that may be asked in Chemistry exams for Grade 11 as a result. These questions were created by our knowledgeable faculty for standard 11 Chemistry based on the questions that appeared most frequently in last year’s tests. The solutions have been written in a way that will make them simple to grasp and will aid students in grade 11 in understanding the topics.
Class 11 Chemistry Books
Importance Class 11 Chemistry Case Study Questions
Class 11 Chemistry Case Study Questions are pivotal in building a strong foundation for future studies in chemistry. They help in:
- Analyzing Problem-solving Skills: These questions test your ability to apply theoretical knowledge in practical situations.
- Developing Critical Thinking: Encouraging logical reasoning and systematic approach to complex problems.
- Enhancing Conceptual Understanding: Making connections between different areas of chemistry.
Study Strategies for Class 11 Chemistry
Time management.
Managing time effectively is key to success. Tips include:
- Creating a Study Schedule: Allocating time for each topic.
- Prioritizing Topics: Focusing on challenging areas first.
Effective Note-taking
Taking effective notes enhances understanding. Methods include:
- Summarizing Information: Writing concise summaries of key points.
- Using Visual Aids: Such as diagrams and charts.
Preparing for Class 11 Chemistry Case Study Questions
Question analysis.
Understanding the question is the first step to a correct answer. Techniques include:
- Identifying Keywords: Recognizing the main aspects of the question.
- Understanding the Context: Relating the question to real-world applications.
Solving Strategies
Strategies for solving Class 11 Chemistry Case Study Questions include:
- Breaking Down the Problem: Dividing the question into smaller, manageable parts.
- Applying Relevant Concepts: Utilizing the appropriate concepts and principles.
Class 11 Chemistry Syllabus 2024
Unit I: Some Basic Concepts of Chemistry ( 18 Periods )
General Introduction: Importance and scope of Chemistry. Nature of matter, laws of chemical combination, Dalton’s atomic theory: concept of elements, atoms and molecules. Atomic and molecular masses, mole concept and molar mass, percentage composition, empirical and molecular formula, chemical reactions, stoichiometry and calculations based on stoichiometry.
Unit II: Structure of Atom ( 20 Periods )
Discovery of Electron, Proton and Neutron, atomic number, isotopes and isobars. Thomson’s model and its limitations. Rutherford’s model and its limitations, Bohr’s model and its limitations, concept of shells and subshells, dual nature of matter and light, de Broglie’s relationship, Heisenberg uncertainty principle, concept of orbitals, quantum numbers, shapes of s, p and d orbitals, rules for filling electrons in orbitals – Aufbau principle, Pauli’s exclusion principle and Hund’s rule, electronic configuration of atoms, stability of half-filled and completely filled orbitals.
Unit III: Classification of Elements and Periodicity in Properties ( 12 Periods )
Significance of classification, brief history of the development of periodic table, modern periodic law and the present form of periodic table, periodic trends in properties of elements -atomic radii, ionic radii, inert gas radii, Ionization enthalpy, electron gain enthalpy, electronegativity, valency. Nomenclature of elements with atomic number greater than 100.
Unit IV: Chemical Bonding and Molecular Structure ( 20 Periods )
Valence electrons, ionic bond, covalent bond, bond parameters, Lewis’s structure, polar character of covalent bond, covalent character of ionic bond, valence bond theory, resonance, geometry of covalent molecules, VSEPR theory, concept of hybridization, involving s, p and d orbitals and shapes of some simple molecules, molecular orbital theory of homonuclear diatomic molecules (qualitative idea only), Hydrogen bond.
Unit VI: Chemical Thermodynamics ( 23 Periods )
Concepts of System and types of systems, surroundings, work, heat, energy, extensive and intensive properties, state functions. First law of thermodynamics -internal energy and enthalpy, heat capacity and specific heat, measurement of ΔU and ΔH, Hess’s law of constant heat summation, enthalpy of bond dissociation, combustion, formation, atomization, sublimation, phase transition, ionization, solution and dilution. Second law of Thermodynamics (brief introduction) Introduction of entropy as a state function, Gibb’s energy change for spontaneous and non- spontaneous processes, criteria for equilibrium. Third law of thermodynamics (brief introduction).
Unit VII: Equilibrium ( 20 Periods )
Equilibrium in physical and chemical processes, dynamic nature of equilibrium, law of mass action, equilibrium constant, factors affecting equilibrium – Le Chatelier’s principle, ionic equilibrium- ionization of acids and bases, strong and weak electrolytes, degree of ionization, ionization of poly basic acids, acid strength, concept of pH, hydrolysis of salts (elementary idea), buffer solution, Henderson Equation, solubility product, common ion effect (with illustrative examples).
Unit VIII: Redox Reactions ( 09 Periods )
Concept of oxidation and reduction, redox reactions, oxidation number, balancing redox reactions, in terms of loss and gain of electrons and change in oxidation number, applications of redox reactions.
Unit XII: Organic Chemistry -Some Basic Principles and Techniques ( 20 Periods )
General introduction, methods of purification, qualitative and quantitative analysis, classification and IUPAC nomenclature of organic compounds. Electronic displacements in a covalent bond: inductive effect, electromeric effect, resonance and hyper conjugation. Homolytic and heterolytic fission of a covalent bond: free radicals, carbocations, carbanions, electrophiles and nucleophiles, types of organic reactions.
Unit XIII: Hydrocarbons ( 18 Periods )
Classification of Hydrocarbons
Aliphatic Hydrocarbons:
Alkanes – Nomenclature, isomerism, conformation (ethane only), physical properties, chemical reactions including free radical mechanism of halogenation, combustion and pyrolysis.
Alkenes – Nomenclature, the structure of double bond (ethene), geometrical isomerism, physical properties, methods of preparation, chemical reactions: addition of hydrogen, halogen, water, hydrogen halides (Markovnikov’s addition and peroxide effect), ozonolysis, oxidation, mechanism of electrophilic addition.
Alkynes – Nomenclature, the structure of triple bond (ethyne), physical properties, methods of preparation, chemical reactions: acidic character of alkynes, addition reaction of – hydrogen, halogens, hydrogen halides and water.
Aromatic Hydrocarbons: Introduction, IUPAC nomenclature, benzene: resonance, aromaticity, chemical properties: mechanism of electrophilic substitution. Nitration, sulphonation, halogenation, Friedel Craft’s alkylation and acylation, directive influence of the functional group in monosubstituted benzene. Carcinogenicity and toxicity.
FAQs about Class 11 Chemistry Case Studies
What is the best website for a case study of chemistry class 11 .
studyrate.in is the best website for Class 11 Chemistry Case Study Questions for Board Exams. Here you can find various types of Study Materials, Ebooks, Notes, and much more free of cost.
What are Class 11 Chemistry Case Study Questions?
Class 11 Chemistry Case Study Questions are examination questions that test a student’s ability to apply theoretical concepts to practical scenarios. They often include real-world situations, requiring critical thinking and problem-solving skills.
Why are Class 11 Chemistry Case Study Questions important?
These questions help in evaluating a student’s understanding of key concepts and their ability to apply them in real-life situations. They encourage analytical thinking, enhance conceptual knowledge, and prepare students for advanced studies.
How can I prepare for Class 11 Chemistry Case Study Questions?
Preparation involves understanding core concepts, practicing regularly, utilizing resources like textbooks and online platforms, and developing problem-solving strategies. Time management and effective note-taking also play vital roles.
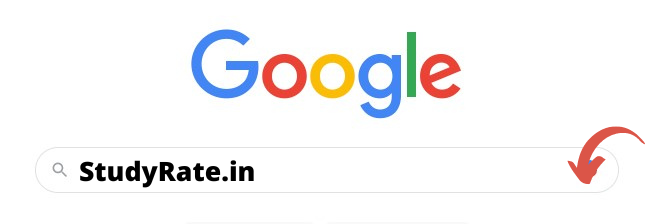
You Might Also Like
50+ jee mains mcq questions structure of atom with solutions, 50+ neet mcq questions chemical bonding and molecular structure with solutions, class 11 physics case study questions chapter 14 oscillations, leave a reply cancel reply.
Save my name, email, and website in this browser for the next time I comment.
This site uses Akismet to reduce spam. Learn how your comment data is processed .
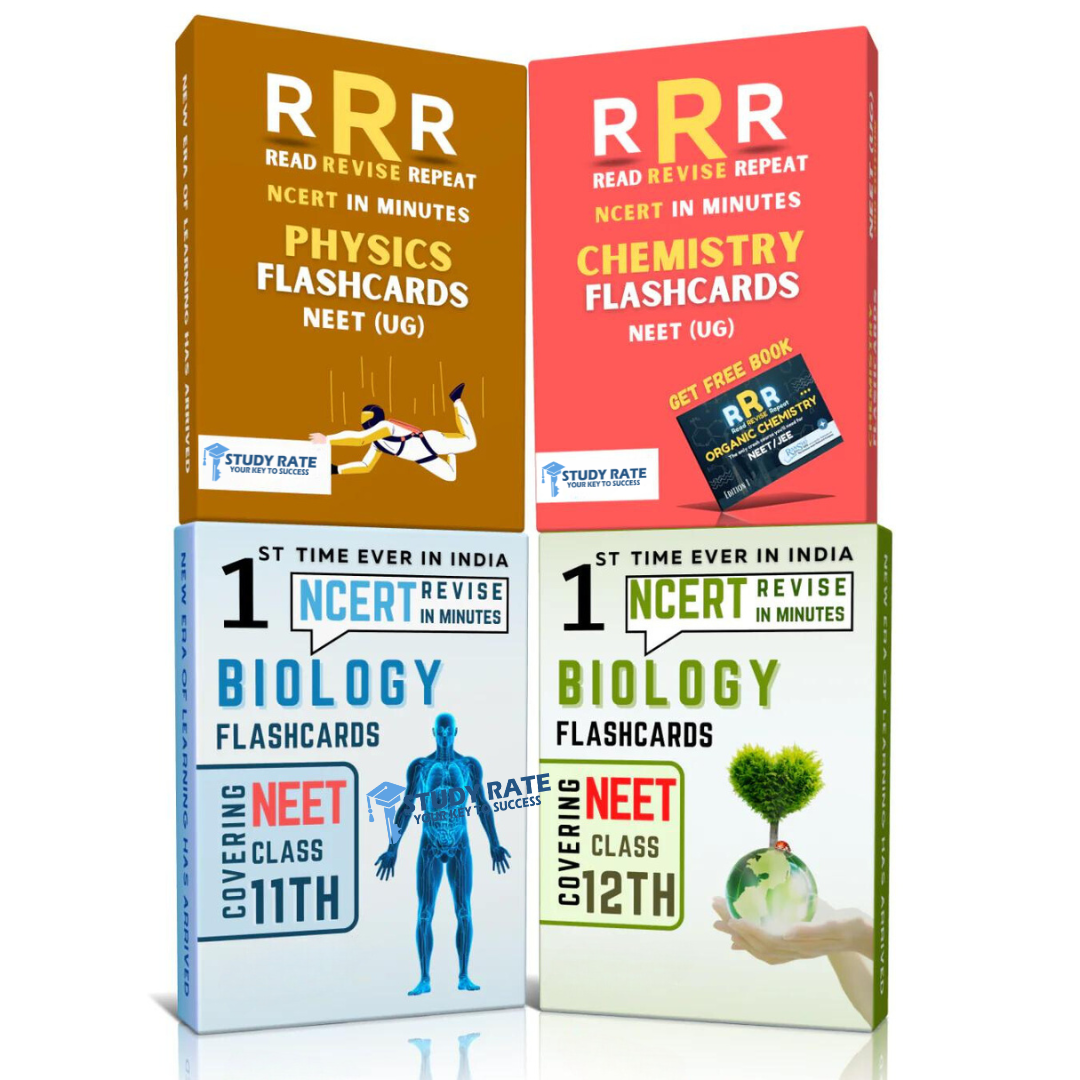
The Topper Combo Flashcards
- Contains the Latest NCERT in just 350 flashcards.
- Colourful and Interactive
- Summarised Important reactions according to the latest PYQs of NEET(UG) and JEE
No thanks, I’m not interested!
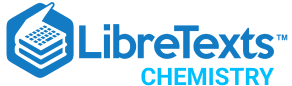
- school Campus Bookshelves
- menu_book Bookshelves
- perm_media Learning Objects
- login Login
- how_to_reg Request Instructor Account
- hub Instructor Commons
- Download Page (PDF)
- Download Full Book (PDF)
- Periodic Table
- Physics Constants
- Scientific Calculator
- Reference & Cite
- Tools expand_more
- Readability
selected template will load here
This action is not available.
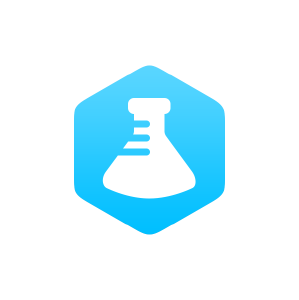
4: Chemical Bonding
- Last updated
- Save as PDF
- Page ID 435632
- 4.1: Lewis structures
- 4.2: Covalent bonding and electronegativity
- 4.3: Valence Bond Theory
- 4.4: Molecular Structure and Polarity
- 4.5: Atomic Structure
myCBSEguide
- Class 11 Chemistry Case...
Class 11 Chemistry Case Study Questions
Table of Contents
myCBSEguide App
Download the app to get CBSE Sample Papers 2023-24, NCERT Solutions (Revised), Most Important Questions, Previous Year Question Bank, Mock Tests, and Detailed Notes.
Are you perplexed? Are you having trouble finding different Class 11 Chemistry Case Study Questions? If the answer is affirmative! You’ve arrived at the perfect place. The myCBSEguide App offers case study questions for CBSE class 11 Chemistry.
CBSE class 11 Chemistry students have always been under a lot of pressure to perform well in their board exams. This pressure has increased in recent years, with the inclusion of case study questions and other Higher Order Thinking Skills Questions. myCBSEguide provides with all the resources that class 11 Chemistry students need to prepare for their class 11 Chemistry exams. This includes sample papers, question banks, case study questions and revision notes prepared by experienced teachers.
The logical reason for Chemistry
Higher Secondary is the most important stage of schooling because it is at this point that specialized discipline-based, content-oriented courses are introduced. Students reach this stage after completing ten years of general education and choose Chemistry to pursue a career in basic sciences or professional courses such as medicine, engineering, or technology, as well as tertiary study courses in applied science and technology. As a result, learners must be provided with a sufficient conceptual background in Chemistry in order to be prepared to meet the challenges of academic and professional courses after high school.
Chemistry in Class 11
Chemistry studies the composition, structure, properties, and reactions of matter. Chemistry is included as one of the major disciplines in CBSE class 11 for a variety of reasons.
- First and foremost, chemistry is essential for understanding the basic structure and composition of matter.
- In addition, chemistry plays a critical role in many other disciplines, such as biology, environmental science, and engineering.
- Furthermore, chemistry is a fascinating subject in its own right, and studying it can provide a deeper understanding of the world around us.
- Finally, chemistry is a practical subject that can be used in everyday life, and it is therefore important for students to have a good understanding of it.
Integrating Case Study Questions in Chemistry for Class 11
Case study questions can be a great way to integrate real-world applications of chemistry into your class 11 curriculum. By posing questions that require students to analyze and apply their knowledge of chemical concepts, you can help them to see how those concepts are relevant to their lives outside of the classroom. Additionally, case study questions can be used to spark lively classroom discussion and debate.
Sample Class 11 Case Study Questions
The recent addition of Case Study Questions in Chemistry for Class 11 by CBSE has been welcomed by students and teachers alike. In Class 11 Chemistry, the case study approach is seen as an engaging and effective way to learn, and the questions included in Class 11 Chemistry is designed to challenge and stimulate students’ thinking. Class 11 Chemistry Case Study Questions cover a wide range of topics, from the basics of atomic structure to more complex concepts such as chemical reactions and equilibrium. The addition of this resource is sure to enhance the learning experience for Class 11 Chemistry students of all abilities while solving Class 11 Chemistry Case Study Questions.
myCBSEguide is the only app that provides a wide range of Class 11 Chemistry Case Study Questions curated by expert teachers. By providing access to this wealth of resources, myCBSEguide makes it easy for Class 11 Chemistry students to learn about and understand complex chemical concepts. In addition, the app’s comprehensive collection of Class 11 Chemistry Case Study Questions covers all major topics in the subject, making it an invaluable resource for students preparing for Class 11 Chemistry exams.
Have a look at few samples of Class 11 Chemistry Case Study Questions for better understanding.
Class 11 Chemistry Case Study Question 1
Read the passage and answer the following question: In 1830, Michael Faraday showed that if electricity is passed through a solution of an electrolyte, chemical reactions occurred at the electrodes, which resulted in the liberation and deposition of matter at the electrodes. In mid-1850s Faraday began to study electrical discharge in partially evacuated tubes, known as cathode ray discharge tubes. When sufficiently high voltage is applied across the electrodes, current starts flowing through a stream of particles moving in the tube from the negative electrode to the positive electrode. These were called cathode rays or cathode ray particles. J.J. Thomson measured the ratio of electrical charge (e) to the mass of the electron (m e ) by using a cathode ray tube and applying electrical and magnetic fields perpendicular to each other as well as to the path of electrons. A positively charged particle was characterised in 1919. Later, a need was felt for the presence of an electrically neutral particles as one of the constituents of the atom.
In this question, a statement of assertion followed by a statement of reason is given. Choose the correct answer out of the following choices
- Assertion and reason both are correct statements and reason is the correct explanation for assertion.
- Assertion and reason both are correct statements and reason is not the correct explanation for assertion.
- Assertion is the correct statement but reason is wrong statement.
- Assertion is the wrong statement but reason is correct statement.
- Assertion: The cathode rays start from cathode and move towards the anode. Reason: In the absence of electrical or magnetic field, cathode rays travel in straight lines.
- Assertion: Thomas argued that the lighter the particle, greater the deflection. Reason: Deflection depends upon the mass of the particle.
- Assertion: Television picture tubes are anode ray tubes. Reason: Electrons are the basic constituent of all the atoms.
- Assertion: The charge to mass ratio of the particles depends on the gas from which these originate. Reason: The smallest and lightest positive ion was obtained from hydrogen and was called proton.
Assertion: A cathode ray tube is made of glass containing two thin pieces of metal electrodes. Reason: The value of e/m e is 2.758820 × 10 11 C kg -11 .
Answer Key:
- (b) Assertion and reason both are correct statements and reason is not the correct explanation for assertion.
- (a) Assertion and reason both are correct statements and reason is the correct explanation for assertion.
- (d) Assertion is the wrong statement but reason is correct statement.
- (c) Assertion is the correct statement but reason is wrong statement.
Class 11 Chemistry Case Study Question 2
Read the passage and answer the following question: The existing large number of organic compounds and their ever-increasing numbers has made it necessary to classify them on the basis of their structures. Organic compounds are broadly classified as open-chain compounds which are also called aliphatic compounds. Aliphatic compounds further classified as homocyclic and heterocyclic compounds. Aromatic compounds are special types of compounds. Alicyclic compounds, aromatic compounds may also have heteroatom in the ring. Such compounds are called heterocyclic aromatic compounds. Organic compounds can also be classified on the basis of functional groups, into families or homologous series. The members of a homologous series can be represented by general molecular formula and the successive members differ from each other in molecular formula by a –CH 2 unit.
In these questions, a statement of assertion followed by the statement of reason is given. Choose the correct answer out of the following choices
- Assertion: Tetrahydrofuran is aliphatic compounds Reason: Sometimes atoms other than carbon are also present in the ring known as heterocyclic.
- Assertion: Hydroxyl group (–OH) is a functional group. Reason: The functional group is defined as an atom or group of atoms joined in a specific manner with characteristic chemical properties of the organic compounds.
- Assertion: Non-benzenoid compound is a classification as the alicyclic compound. Reason: Aniline is a benzenoid compound.
- Assertion: H 2 C=CH 2 is a condensed structural formula. Reason: Condensed structural formula is represented by omitting some or all of the dashes representing covalent bonds.
Assertion: Cyclic compound is classified as a carbocyclic and heterocyclic compound. Reason: Thiophene is a homocyclic compound.
Class 11 Chemistry Case Study Question 3
Read the passage and answer the following questions: A large number of orbitals are possible in an atom. Qualitatively these orbitals can be distinguished by their size, shape and orientation. An orbital of smaller size means there is more chance of finding the electron near the nucleus. Similarly, shape and orientation mean that there is more probability of finding the electron along with certain directions than along others. The principal quantum number determines the size and to large extent the energy of the orbital. Azimuthal quantum number, ‘l’ is also known as orbital angular momentum or subsidiary quantum number. It defines the three-dimensional shape of the orbital. Each shell consists of one or more subshells or sub-levels. The number of sub-shells in a principal shell is equal to the value of n. Magnetic orbital quantum number. ‘ml ’ gives information about the spatial orientation of the orbital with respect to a standard set of co-ordinate axis. The fourth quantum number is known as the electron spin quantum number (m s ). An electron spins around its own axis, much in a similar way as the earth spins around its own axis while revolving around the sun. In these questions, a statement of assertion followed by the statement of reason is given. Choose the correct answer out of the following choices:
- Assertion: Each orbital is designated by three quantum numbers labelled as n, l and m l . Reason: ‘n’ is a positive integer with value of n = 1,2,3.
- Assertion: The principal quantum number identifies the shell. Reason: Size of an orbital decrease with the increase of principal quantum number ‘n’.
- Assertion: For n = 2, the possible value of l can be 0 and 1. Reason: For a given value of n, l can have n values ranging from 0 to n – 1.
- Assertion: Each orbital in an atom, is defined by a set of values for n, l and m l . Reason: m l designates the orientation of the orbital. OR Assertion: Spin quantum numbers m s can take the values of +½ or –½. Reason: Two spin states of the electron and are normally represented by two arrows, ↑ (spin down) and ↓ (spin up).
- (b) Assertion and reason both are correct statements and reason is not the correct explanation for assertion. OR (c) Assertion is the correct statement but reason is wrong statement.
Significance of Class 11 Chemistry case study questions
Case study questions in Class 11 Chemistry are important because:
- They help Class 11 Chemistry students understand basic chemistry facts and concepts while keeping the excitement alive.
- It prepares Class 11 Chemistry students to study chemistry in tertiary courses such as medicine, engineering, and technology. • introduce students to a variety of emerging new areas of chemistry and inform them of their importance in future studies as well as their applications in various fields of chemical science and technology.
- It prepares Class 11 Chemistry students to face a variety of health, nutrition, environmental, population, weather, industry, and agriculture challenges.
- It also improves Class 11 Chemistry students’ problem-solving abilities.
- It helps in familiarizing Class 11 Chemistry students with various industrial processes as well as their technological applications.
Glancing the Class 11 Chemistry syllabus
The revised and updated Class 11 Chemistry syllabus is built on a disciplinary approach with rigour and depth, while keeping the Class 11 Chemistry syllabus light and comparable to the worldwide level. Greater emphasis has been placed in the Class 11 Chemistry syllabus on the use of new nomenclature, symbols, and formulations, the teaching of fundamental concepts, the application of concepts in chemistry to industry/technology, logical sequencing of units, the removal of obsolete content and repetition, and so on.
CBSE Class 11 Chemistry (Code No. 043) Syllabus (2022-23)
myCBSEguide: Balanced equation for Class 11 Chemistry students
For Class 11 Chemistry students, myCBSEguide is always a balanced equation. It includes a plethora of class 11 chemistry case study questions to help students better understand the ideas in Class 11 Chemistry. Class 11 chemistry case study questions are intended to assess students’ comprehension while also providing a platform for them to strengthen their problem-solving abilities. myCBSEguide also provides a number of other tools that students can utilise to deepen their grasp of the subject. Additionally, the answer keys and explanations provided by myCBSEguide are always clear and concise, making it an excellent tool for students who want to improve their grades. So, what are you waiting for? Get myCBSEguide today and start studying for your future.
Test Generator
Create question paper PDF and online tests with your own name & logo in minutes.
Question Bank, Mock Tests, Exam Papers, NCERT Solutions, Sample Papers, Notes
Related Posts
- Competency Based Learning in CBSE Schools
- Class 11 Physical Education Case Study Questions
- Class 11 Sociology Case Study Questions
- Class 12 Applied Mathematics Case Study Questions
- Class 11 Applied Mathematics Case Study Questions
- Class 11 Mathematics Case Study Questions
- Class 11 Biology Case Study Questions
- Class 12 Physical Education Case Study Questions
Leave a Comment
Save my name, email, and website in this browser for the next time I comment.
- Publications
- Conferences & Events
- Professional Learning
- Science Standards
- Awards & Competitions
- Daily Do Lesson Plans
- Free Resources
- American Rescue Plan
- For Preservice Teachers
- NCCSTS Case Collection
- Partner Jobs in Education
- Interactive eBooks+
- Digital Catalog
- Regional Product Representatives
- e-Newsletters
- Bestselling Books
- Latest Books
- Popular Book Series
- Prospective Authors
- Web Seminars
- Exhibits & Sponsorship
- Conference Reviewers
- National Conference • Denver 24
- Leaders Institute 2024
- National Conference • New Orleans 24
- Submit a Proposal
- Latest Resources
- Professional Learning Units & Courses
- For Districts
- Online Course Providers
- Schools & Districts
- College Professors & Students
- The Standards
- Teachers and Admin
- eCYBERMISSION
- Toshiba/NSTA ExploraVision
- Junior Science & Humanities Symposium
- Teaching Awards
- Climate Change
- Earth & Space Science
- New Science Teachers
- Early Childhood
- Middle School
- High School
- Postsecondary
- Informal Education
- Journal Articles
- Lesson Plans
- e-newsletters
- Science & Children
- Science Scope
- The Science Teacher
- Journal of College Sci. Teaching
- Connected Science Learning
- NSTA Reports
- Next-Gen Navigator
- Science Update
- Teacher Tip Tuesday
- Trans. Sci. Learning
MyNSTA Community
- My Collections
The Name’s Bond, Chemical Bond
Agent Forever Chemical
By Katie McShea, Kari Fleuriet, Fatmah Alamoudi, Deana Jaber
Share Start a Discussion
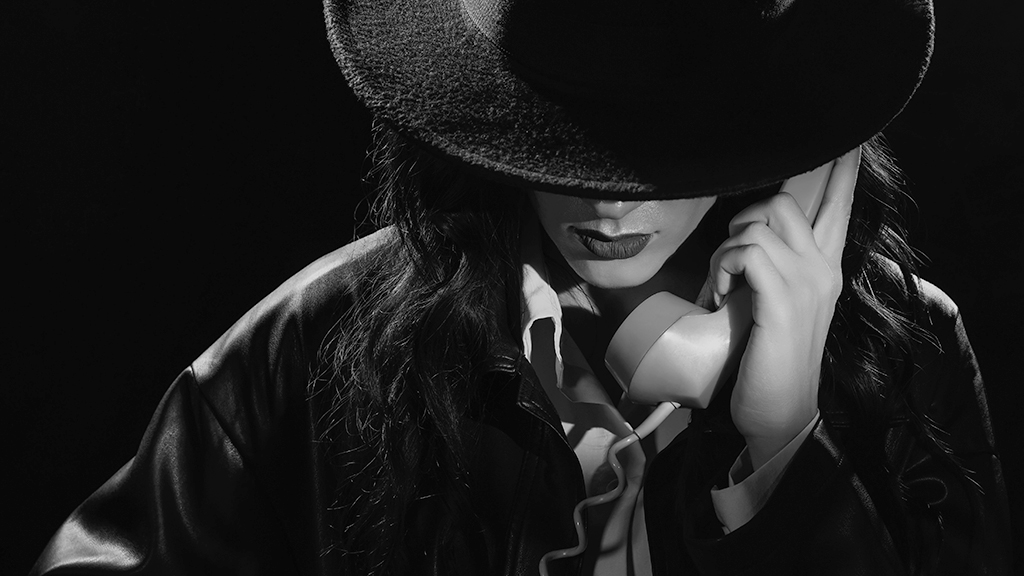
This case study is designed to help students better understand chemical bonds by introducing them to perfluoroalkyl and polyfluoroalkyl substances (PFAS), often referred to as “forever chemicals.” The unique structure of these substances renders them practically non-biodegradable due to the presence of the carbon-fluorine (C-F) bond, the strongest bond in organic chemistry. The case tells the story of “Kayla Bond,” a biochemistry major, who learns of her mother’s diagnosis of type 2 diabetes and wonders whether it could be a consequence of PFAS contamination in their hometown water supply. To find out whether there is a connection, Kayla investigates what makes the C-F bond so strong, and in the process deepens her understanding of concepts such as electronegativity, atomic radius, bond length, dipole moment, and bond enthalpy. Originally developed for biology, biochemistry, and health science majors in an introductory chemistry course, the case could also be adapted for general chemistry or environmental science courses.
Download Case
Date Posted
- Recognize and describe the different types of chemical bonds (ionic, polar covalent, and pure covalent).
- Classify bonds based on difference in electronegativity values.
- Explain the concepts of electronegativity, dipole moment, atomic radius, bond length, and bond enthalpy.
- Identify the periodic trends for electronegativity and atomic radius.
- Identify bonds that have dipole moment.
- Predict bond strength based on atomic radius, bond length, and bond enthalpy.
PFAS; chemical bonds; perfluoroalkyl; polyfluoroalkyl; forever chemicals; carbon-fluorine bond; covalent bond; electronegativity; bond strength; Teflon; Dupont; 3M
Subject Headings
EDUCATIONAL LEVEL
High school, Undergraduate lower division
TOPICAL AREAS
TYPE/METHODS
Teaching Notes & Answer Key
Teaching notes.
Case teaching notes are protected and access to them is limited to paid subscribed instructors. To become a paid subscriber, purchase a subscription here .
Teaching notes are intended to help teachers select and adopt a case. They typically include a summary of the case, teaching objectives, information about the intended audience, details about how the case may be taught, and a list of references and resources.
Download Notes
Answer Keys are protected and access to them is limited to paid subscribed instructors. To become a paid subscriber, purchase a subscription here .
Download Answer Key
Materials & Media
Supplemental materials, you may also like.
Web Seminar
Join us on Thursday, June 13, 2024, from 7:00 PM to 8:00 PM ET, to learn about the science and technology of firefighting. Wildfires have become an e...
Join us on Thursday, October 10, 2024, from 7:00 to 8:00 PM ET, for a Science Update web seminar presented by NOAA about climate science and marine sa...
Secondary Pre-service Teachers! Join us on Monday, October 21, 2024, from 7:00 to 8:15 PM ET to learn about safety considerations for the science labo...
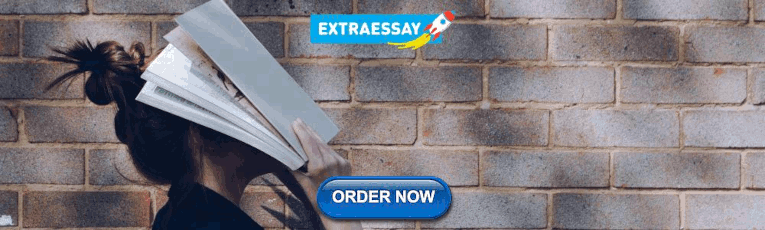
IMAGES
VIDEO
COMMENTS
It is more than dots and lines on paper—it represents the valence electrons forming chemical bonds and non-bonding pairs, both of which dictate the drug's reactivity and interaction with the body's biomolecules. Case Study Questions: Explain the concept of a Lewis structure. How can understanding chemical bonding aid in drug development?
3) In the formation of a molecule, only the outer shell electrons take part in chemical combination and they are known as …. (a) Backscattered electrons. (b) valence electrons. (c) Primary electrons. (d) Secondary electrons. Ans- b) valence electrons. 4) In the CH4 molecule there are … valence electrons available for bonding.
Hope the information shed above regarding Case Study and Passage Based Questions for Class 11 Chemistry Chapter 4 Chemical Bonding and Molecular Structure with Answers Pdf free download has been useful to an extent. If you have any other queries about CBSE Class 11 Chemistry Chemical Bonding and Molecular Structure Case Study and Passage Based Questions with Answers, feel free to comment below ...
To answer such questions different theories and concepts have been put forward from time to time. In this fourth unit of class 11 chemistry, we can answer the above questions by learning Kössel-Lewis approach, Valence Shell Electron Pair Repulsion (VSEPR) Theory, Valence Bond (VB) Theory and Molecular Orbital (MO) Theory.
Before beginning the case study, complete the five questions below, which review important topics you have previously learned about in class: (1) atomic structure, (2) electron configuration, and (3) valence, core, and outer electrons. ... "Let's start by exploring chemical bonds," said Dr. Johnson "Chemical bonds are formed between ...
1. Introduction. Bonding is what separates chemistry from physics. If the understanding of atoms and their component particles belongs primarily to the realm of physics, then chemistry is concerned with the aggregation of atoms into chemical entities held together by bonds.If science is a language, and atoms are the letters, bonding is the mechanism by which the letters are combined into words.
Knowledge of tautological concepts (chemical bond, ionic bond, covalent bond, ionic compound, covalent compound): With the exception of the PuS in the case of the chemical bond concept, correct responses ranged from 84 to 100%, with 17 out of the 20 cases being above 90%. In the case of the chemical bond concept (where as correct we assumed the ...
Case Study Questions for Class 11 Chemistry Chapter 4 Chemical Bonding and Molecular Structure Case Study/Passage Based Questions: Question 1: Lewis dot structure of HCN molecule is given below. Answer questions on the basis of this structure. (i) How many electrons are involved in the sigma bond between the carbon atom and the nitrogen atom?(A) … Continue reading Case Study Questions for ...
Research has revealed that students may have a wide range of alternative conceptions relating to aspects of chemical bonding - although many of the common ideas fit into a clear pattern: a coherent alternative framework. ... D. F. (2000) Learning about atoms, molecules, and chemical bonds: a case study of multiple-model use in grade 11 ...
Chemical bonding is undoubtedly one of the key concepts in chemistry and one of the most fundamental. It is also one of the areas in the physical sciences where understanding is developed through diverse models—which are in turn built on a range of physical principles—and where students are expected to interpret a disparate range of symbolic representations of chemical bonds (Taber and ...
Abstract. This case study presents the story of "Nick," a student who has been assigned the task of writing a research paper describing the fundamentals of chemical bonds and how they relate to human life. When Nick experiences difficulty remembering information about the different types of chemical bonds, he turns to his tutor, Josh, for help.
A report from the journal Science Education focusing on the Harrison and Treagust article Learning about Atoms, Molecules, and Chemcial Bonds: A Case Study. Learning about Atoms, Molecules, and Chemical Bonds: A Case Study of Multiple-Model Use | Journal of Chemical Education
QB365 provides a detailed and simple solution for every Possible Case Study Questions in Class 11 Chemistry Subject - Chemical Bonding and Molecular Structure, CBSE. It will help Students to get more practice questions, Students can Practice these question papers in addition to score best marks.
Answers. Que No. 1 Answers-. 1.) Covalent bonds are of following three types:1)Single covalent bond 2) Double covalent bond 3)Triple covalent bond. 2.) The covalent compounds are said to be polarwhen the shared pair of electrons are not at equaldistance between the two atoms and Covalent compounds are non-polar when sharedpair of electron (s ...
Class 11 Chemistry Case Study Questions Chapterwise PDF Download. Class 11 Chemistry is divided into intriguing chapters, each unfolding a different aspect of chemical science. ... Chemical Bonding and Molecular Structure: 20: 7: 5. Chemical Thermodynamics: 23: 9: 6. Equilibrium: 20: 7: 7. Redox Reactions: 9: 4: 8. Organic Chemistry: Some basic ...
General, Organic, and Biochemistry with Problems, Case Studies, and Activities ... Chemical Bonding Expand/collapse global location 4: Chemical Bonding Last updated; Save as PDF Page ID 435632 ... 4.3: Valence Bond Theory; 4.4: Molecular Structure and Polarity; 4.5: Atomic Structure ...
Class 11 Chemistry Case Study Question 1. Read the passage and answer the following question: In 1830, Michael Faraday showed that if electricity is passed through a solution of an electrolyte, chemical reactions occurred at the electrodes, which resulted in the liberation and deposition of matter at the electrodes.
This case study is designed to help students better understand chemical bonds by introducing them to perfluoroalkyl and polyfluoroalkyl substances (PFAS), often referred to as "forever chemicals.". The unique structure of these substances renders them practically non-biodegradable due to the presence of the carbon-fluorine (C-F) bond, the ...