Your browser does not support javascript. Some site functionality may not work as expected.
- Government Reports
- Books & eBooks
- Citation Help
- Writing a Literature Review
- University of Washington Libraries
- Library Guides
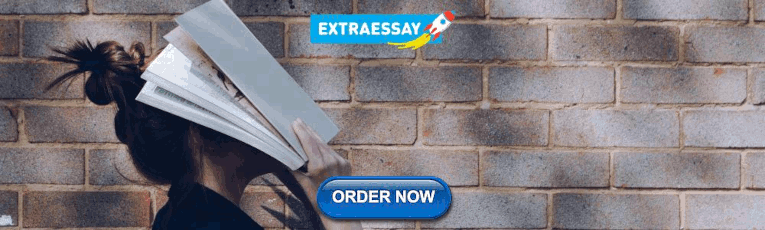
Physics: Writing a Literature Review
Literature reviews.
A literature review surveys scholarly articles, books and other sources (e.g. dissertations, conference proceedings) relevant to a particular issue, area of research, or theory, providing a description, summary, and critical evaluation of each work.
- Provide context for a research paper
- Explore the history and development of a topic
- Examine the scholarly conversation surrounding the topic
- Shows relationships between studies
- Examines gaps in research on the topic
Components
Similar to primary research, development of the literature review requires four stages:
- Problem formulation—which topic or field is being examined and what are its component issues?
- Literature search—finding materials relevant to the subject being explored
- Data evaluation—determining which literature makes a significant contribution to the understanding of the topic
- Analysis and interpretation—discussing the findings and conclusions of pertinent literature
Conducting a Literature Review
1. choose a topic. define your research questions..
Your literature review should be guided by a central research question. Remember, it is not a collection of loosely related studies in a field but instead represents background and research developments related to a specific research question, interpreted and analyzed by you in a synthesized way.
- Make sure your research question is not too broad or too narrow. Is it manageable?
- Begin writing down terms that are related to your question. These will be useful for searches later.
- If you have the opportunity, discuss your topic with your professor.
2. Decide on the scope of your review.
- How many studies do you need to look at?
- How comprehensive should it be?
- How many years should it cover?
Tip: This may depend on your assignment. How many sources does the assignment require?
3. Select the databases you will use to conduct your searches.
Make a list of the databases you will search.
Where to find databases:
- Find Databases by Subject
- T he Find Articles tab of this guide
This page contains a list of the most relevant databases for most Physics research.
4. Conduct your searches and find the literature. Keep track of your searches!
- Review the abstracts of research studies carefully. This will save you time.
- Write down the searches you conduct in each database so that you may duplicate them if you need to later (or avoid dead-end searches that you'd forgotten you'd already tried).
- Use the bibliographies and references of research studies you find to locate others.
- Ask your professor or a librarian if you are missing any key works in the field.
5. Review the Literature
Some questions to help you analyze the research:
- What was the research question of the study you are reviewing? What were the authors trying to discover?
- Was the research funded by a source that could influence the findings?
- What were the research methodologies? Analyze its literature review, the samples and variables used, the results, and the conclusions. Does the research seem to be complete? Could it have been conducted more soundly? What further questions does it raise?
- If there are conflicting studies, why do you think that is?
- How are the authors viewed in the field? Has this study been cited?; if so, how has it been analyzed?
Tips:
- Again, review the abstracts carefully.
- Keep careful notes so that you may track your thought processes during the research process.
Chat with a UW Librarian
- << Previous: Citation Help
- Last Updated: Apr 7, 2024 3:23 PM
- URL: https://guides.lib.uw.edu/bothell/physics

Quick Links:

- Where do I find physics resources in the library?
- What if I can't find what I'm looking for?
- How do I know if my source is a "scholarly" source?
- How do I paraphrase something?
Literature Review Basics
- Literature Review Step-by-Step
- Common Questions about Literature Reviews
- How do I craft a basic citation?
- What is citation tracing?
- How do I use Zotero for citation management?
- Who do I contact for help?
This video will provide a short introduction to literature reviews.
Steps For Writing a Literature Review
Recommended steps for writing a literature review:
- Review what a literature review is, and is not
- Review your assignment and seek clarification from your instructor if needed
- Narrow your topic
- Search and gather literature resources.
- Read and analyze literature resources
- Write the literature review
- Review appropriate Citation and Documentation Style for your assignment and literature review
Common Questions
What is a literature review?
A literature review is a type of scholarly, researched writing that discusses the already published information on a narrow topic .
What is the purpose of a writing literature review?
Writing a literature review improves your personal understanding of a topic, and demonstrates your knowledge and ability to make connections between concepts and ideas. The literature review is a service to your reader, summarizing past ideas about a topic, bringing them up to date on the latest research, and making sure they have all any background information they need to understand the topic.
What is "the literature"?
This already published information- called the literature- can be from primary information sources such as speeches, interviews, and reports, or from secondary information sources such as peer-reviewed journal articles, dissertations, and books. These type of sources are probably familiar to you from previous research projects you’ve done in your classes.
Is a literature review it's own paper?
You can write a literature review as a standalone paper , or as part of a larger research paper . When a standalone paper, the literature review acts as a summary, or snapshot, of what has been said and done about a topic in the field so far. When part of the a larger paper, a literature review still acts as a snapshot, but the prior information it provides can also support the new information, research, or arguments presented later in the paper.
Does a literature review contain an argument?
No, a literature review does NOT present an argument or new information. The literature review is a foundation that summarizes and synthesizes the existing literature in order for you and your readers to understand what has already been said and done about your topic.
- << Previous: How do I paraphrase something?
- Next: How do I craft a basic citation? >>
- Last Updated: Mar 29, 2024 11:34 AM
- URL: https://fhsuguides.fhsu.edu/physics

Doing a literature review using digital tools (with Notion template)
I’ve recently revamped my literature review workflow since discovering Notion . Notion is an organization application that allows you to make various pages and databases. It’s kind of like your own personal wiki- you can link your pages and embed databases into another page, adding filters and sorting them using user-set properties. The databases are what I use the most. I’ve essentially transferred all of my excel sheets into Notion databases and find it much easier to filter and sort things now. In this post, I’ll go through how I do my literature review and share a Notion template that you can use.
I like to organize my literature review using various literature review tools along with two relational Notion databases: a ‘literature tracker’ and a ‘literature notes’ matrix. You can see a flow chart of my literature review process below (it’s inspired by this post by Jenn’s Studious Life and the three pass method for reading papers which I wrote about last week in this post ):

As you can see, this process involves a couple of decision points which helps me focus on the most important papers. This is an iterative process that keeps me up to date on relevant research in my field as I am getting new paper alerts in my inbox most days. I used this method quite successfully to write the literature review for my confirmation report and regularly add to it for the expanded version that will become part of my PhD thesis. In this post, I’ll break down how this works for me and how I implement my Notion databases to synthesise the literature I read into a coherent argument.
You can click on the links below to navigate to a particular section of this article:
The literature search
The literature tracker, the literature synthesis matrix, writing your literature review, iterating your literature review, my literature review notion template, some useful resources.
This is always the first step in building your literature review. There are plenty of resources online all about how to start with your search- I find a mixture of database search tools works for me.
The first thing to do when starting your literature review is to identify some keywords to use in your initial searches. It might be worth chatting to your supervisor to make a list of these and then add or remove terms to it as you go down different research routes. You can use keyword searches relevant to your research questions as well tools that find ‘similar’ papers and look at citation links. I also find that just looking through the bibliographies of literature in your field and seeing which papers are regularly cited gives you a good idea of the core papers in your area (you’ll start recognising the key ones after a while). Another method for finding literature is the snowballing method which is particularly useful for conducting a systematic review.
Here are some digital tools I use to help me find literature relevant to my research questions:
Library building and suggestions
Mendeley was my research management tool of choice prior to when I started using Notion to organize all of my literature and create my synthesis matrix. I still use Mendeley as a library just in case anything happens to my Notion. It’s easy to add new papers to your library using the browser extension with just one click. I like that Mendeley allows you to share your folders with colleagues and that I can export bib.tex files straight from my library into overleaf documents where I’m writing up papers and my thesis. You do need to make sure that all of the details are correct before you export the bib.tex files though as this is taken straight from the information plane. I also like to use the tag function in Mendeley to add more specific identifiers than my folders.
Mendeley is also useful for finding literature related to those in your library- I’ve found quite a few interesting papers through the email updates they send out each week with ‘suggested papers’. You can also browse these suggestions from within Mendeley and use its interface to do initial keyword searches. The key is to just scan the titles and then decide whether it’s worth your time reading the abstract and then the rest of it. It’s easy to get overwhelmed by the sheer amount of papers being published every day so being picky in what you read is important (and something I need to work on more!).

Some similar tools that allow you to build a library and get literature recommendations include Zotero , Researcher , Academia , and ResearchGate . It’s up to you which one you use for your own purposes. One big factor for me when choosing Mendeley was that my supervisor and colleagues use it so it makes it much easier to share libraries with them, so maybe ask your colleagues what they use before settling on one.
Literature databases and keyword alerts
There are a variety of databases out there for finding literature. My go-to is Web of Science as it shows you citation data and has a nice interface. I used this to begin my initial literature search using my keywords.
The other thing you can do with these kinds of tools is set up email alerts to get a list of recent work that has just been published with any keywords you set. These alerts are usually where I find papers to read during journal club with my supervisor. You can customize these emails to what suits you- mine are set to the top 10 most relevant new papers for each keyword weekly and I track around 5 words/phrases. This allows me to stay on top of the most recent literature in my field- I have alerts set up on a variety of services to ensure that I don’t miss anything crucial (and alerts from the ArXiv mean I see preprints too). Again, you need to be picky about what you read from these to ensure that they are very relevant to your research. At this stage, it’s important to spend as little time as possible scanning titles as this can easily become a time suck.

Some of the other tools I have keyword (and author) email alerts set up on are: Scopus , Google Scholar , Dimensions , and ArXiv alerts . I set 10 minutes maximum aside per day to scan through any new email alerts and save anything relevant to me into my literature tracker (which I’ll come to more later).
Literature mapping tools
There are loads of these kinds of tools out there. Literature mapping can be helpful for finding what the seminal papers are in your field and seeing how literature connects. It’s like a huge web and I find these visual interfaces make it much easier to get my head around the relationships between papers. I use two of these tools during the literature search phase of the flowchart: Citation Gecko and Connected Papers .
Citation Gecko builds you a citation tree using ‘seed papers’. You can import these from various reference management software (like Mendeley), bib.tex files or manually search for papers. This is particularly useful if your supervisor has provided you with some core papers to start off with, or you can use the key papers you identified through scanning the bibliographies of literature you read. My project is split into fairly clear ‘subprojects’ so these tools help me see connections between the various things I’m working on (or a lack of them which is good in some ways as it shows I’ve found a clear research gap!).

You can switch between different views and add connecting papers as new seed papers to expand your network. I use this tool from time to time with various different papers associated with my subprojects. It’s helped me make sure I haven’t missed any key papers when doing my literature review and I’ve found it to be fairly accurate, although sometimes more recent papers don’t have any citation data on it so that’s something to bear in mind.
Connected Papers uses a ‘similarity’ algorithm to show paper relationships. This isn’t a citation tree like Citation Gecko but it does also give you prior and derivative works if you want to look at them. All you do is put one of your key papers into the search box and ‘build a graph’. It will then show you related papers, including those which don’t have direct citation links to the key paper. I think this is great for ensuring that you’re not staying inside an insular bubble of the people who all cite each other. It also allows me to see some of the research which is perhaps a bit more tangential to my project and get an overview of where my work sits within the field more broadly.

I like Connected Paper’s key for the generated tree and that it shows where related papers connect between themselves. Again, it’s helpful for ensuring that you haven’t missed a really important work when compiling your literature review and doesn’t just rely on citation links between papers.
This is where I record the details of any paper I come across that I think might be relevant to my PhD. In some ways, it’s very similar to Mendeley but it’s a version that sits within Notion so I have some more customised filtering categories set up, like my ‘status’ field where I track which pass I am on.
Here’s what my literature tracker looks like:

The beauty of Notion is that you can decide which properties you want to record in your database and customize it to your needs. You can sort and filter using these properties including making nested filters and using multiple filters at once. This makes it really easy to find what you’re looking for. For example, say I’m doing my literature review for my ‘FIB etching’ subproject and want to see all of the papers that I marked as relevant to my PhD but haven’t started reading yet. All I need to do is add a couple of filters:

And it filters everything so that I’m just looking at the papers I want to check out. It’s this flexibility that I think really gives Notion the edge when it comes to my literature review process.
The other thing I really like about using Notion rather than excel is that I can add different database views. I especially like using the kanban board view to see where I’m at with my reading workflow:

When I add something to the literature tracker database, I scan the abstract for keywords to add and categorize it in terms of relevant topics. It’s essentially the first pass of the paper, so that involves reading the title, abstract, introduction, section headings, conclusions, and checking the references for anything you recognise. After this is done, I decide whether it’s relevant enough to my PhD to proceed to do a second pass of the paper, at which point I will progress to populating my literature notes database.
Once I’ve decided that I want to do a second pass on a paper, I then add it to the ‘literature notes’ database. This is part of the beauty of Notion: relational databases. I have ‘rollup’ properties set in the literature notes database which shows all of the things I added during my first pass and allows me to filter the matrix using them. You can watch the video below to see exactly how to add a new paper to the ‘notes’ database from the ‘tracker’ database:
During the second pass, I populate the new fields in the ‘notes’ database. These are:
Summary | Objective of study | Key Results | Theory | Materials | Methods | Conclusions | Future work suggested | Critiques | Key connected papers.
I also have various themes/questions/ideas as properties which I add a few notes on for each relevant paper. I then complete my ‘questions for critical engagement’ which are on the entry’s ‘Notes’ page and are stored in the ‘Article Template’. If you want to read more about this process, check out my ‘how to read a scientific paper’ post .
By, doing this I create a synthesis matrix where I can see a breakdown of the key aspects of each paper and can scan down a column to get an overview of all of the papers I have read. For example, if I wanted to see all of the papers about Quantum Point Contacts to get an idea of what previous work has been done so that I can identify my research gap, I can filter using the tag property and can then see the notes I wrote for each entry, broken down by section. I also have tags for my research questions or themes, materials used, experimental techniques, fabrication techniques, and anything else that comes to mind really! The more tags I have for a paper, the easier it is to filter when I want to find a specific thing.
The other property I have included in the literature notes database is ‘Key connected papers’. This is a relation but is within the database itself. So it means that I can link to the page of other papers in the literature matrix. I’ve found this to be useful for connecting to what I call ‘core’ papers. I can also filter using this property, allowing me to see my notes on all of the papers I’ve read that are related to a certain ‘core’ paper. This helps with synthesising all of the information and forming my argument.

For those papers most relevant to my research (the ‘core’ papers) I’ll also do a third pass which involves reimplementing the paper in my own words. This is quite a time-consuming task so not many papers reach this stage, but those which I have done a third pass on are the ones I know really well. My hope is that this will stand me in good stead for my viva. This process also helps me refine my research questions further as I gain a deeper understanding of the field.
I find that writing up a review is extremely intimidating, but having the literature matrix makes this process that bit easier. I won’t go into too many details as there are already loads of resources out there going into the details of writing up a review, but here’s a brief overview of my own process:
Identify your research themes
Using your literature matrix, review each research theme or question and decide which ones you are going to focus on. These will form the different sections of your literature review and help you write your thesis statement(s). You can also think about how your questions link to ensure that you’re telling a coherent story with your review.
Choose and summarize literature related to each theme
For each section, gather up the most important related literature and summarize the key points of each source. A good literature review doesn’t need to cover all the literature out there, just the most significant sources. I try to stick to around 10 or fewer key sources per section.
Critical evaluation of sources
This is where you utilize the ‘questions for critical engagement’. Make sure you evaluate the strengths and weaknesses of the studies you’re writing about. By doing this, you can establish where our knowledge is lacking which will come in helpful later when establishing a research gap.
Analyse each source in relation to other literature
Try to make sure that you are telling a coherent story by linking between your sources. You can go back to the literature matrix here and use it to group similar studies to compare and contrast them. You should also discuss the relevance of the source’s findings in relation to the broader field and core papers.
Situate your research in a research gap
This is where you justify your own research. Using what you have laid out in the rest of the review, show that there is a research gap that you plan to fill and explain how you are going to do that. This should mean that your thesis flows nicely into the next section where you’ll cover the materials and methods you used in your research project.

In some ways, a literature review never really ends. As you can see in the flowchart at the beginning of this post, I regularly update and revise my literature review as well as refining my research questions. At this point in my PhD, I think that most of my research questions are quite well defined, so I’m mostly just adding any newly published work into my review. I don’t spend much time reading literature at the moment but I’m sure I’ll return to it more regularly when I’m in the write-up phase of my PhD. There is a balance to be had between reading and writing for your literature review and actually getting on with your own research!
Here’s the link to my Notion Literature Review Template . You can duplicate it and adapt it however you want, but this should save you some time setting up the initial databases if you’d like to use my method for organizing your own literature review.

Here are some resources on how to do a literature review that I’ve found useful during my PhD:
- The Literature Review: Step-by-Step Guide for Students
- 3 Steps to Save You From Drowning in Your Literature Review
- How to write a literature review
- How to become a literature searching ninja
- Mind the gap
- 7 Secrets to Write a PhD Literature Review The Right Way
If you like my work, I’d love your support!
Share this:
- Click to share on Twitter (Opens in new window)
- Click to share on Facebook (Opens in new window)
- Click to share on LinkedIn (Opens in new window)
11 thoughts on “Doing a literature review using digital tools (with Notion template)”
Thank you so much for your insight and structured process. This will help me a lot kicking off my Master Thesis.
The perfect method to organize the literature that I have read and will read in the future. I am so glad to have found your website, this will save me from thrashing around in the swamp of literature. I was already feeling the limits of my memory when I was doing my master thesis and this will be so helpful during my PhD.
Thank you so much for this detailed post! Lily 🙂
Thank you very much for this. I’m doing my undergrad atm and reading a lot of papers. This seems like an excellent way of tracking everything.
Thank you, you made my beginning less stressful. I like your system and i helped me a lot. I have one question (more might come later), What do you mean by " journal club with my supervisor."
This piece is really really helpful! I started from this one and went through the rest blog writings. I agree on many points with Daisy. I had an unhappy experience of PhD two years ago and now just started a new one in another country. I will take it as an adventure and enjoy it.
This is an AMAZING template. I've found this so helpful for my own workflow. Thank you so much!
I found this post really helpful. Thank you.
thank you very much!
Hi! Thank you very much for posting this guide and sharing your notion template! I do have a question—do you manually enter the references into Notion, or is there any way to speed up the process? Ta x
Leave a Reply Cancel reply
Discover more from notes from the physics lab.
Subscribe now to keep reading and get access to the full archive.
Type your email…
Continue reading
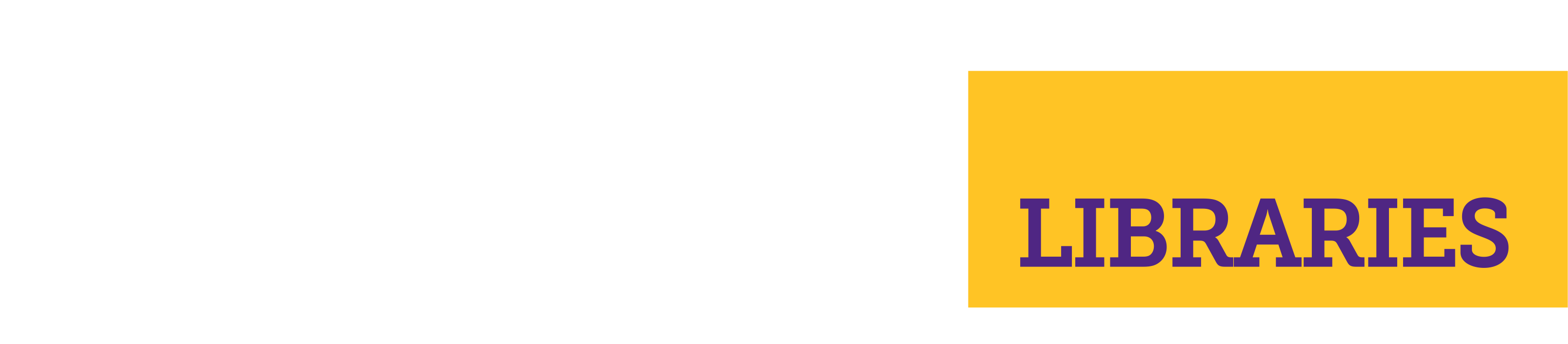
- Joyner Library
- Laupus Health Sciences Library
- Music Library
- Digital Collections
- Special Collections
- North Carolina Collection
- Teaching Resources
- The ScholarShip Institutional Repository
- Country Doctor Museum
Physics and Astronomy: Literature Review
- Find Articles
- Literature Review
- Evaluate Sources
- Citation Guide
Choosing a Topic
Choosing your topic is one of the most important steps for a graduate student, and should be done in consultation with your faculty advisor. Some of the tips presented in the video below can help you get started.
The Literature Review
This tutorial from NCSU gives a good overview of the process of the literature review.
Types of Literature Reviews
Completing Literature Reviews
Links to Further Help You...
- UNC Writing Center Handout for Writing a Lit Review
- Purdue Online Writing Lab Social Work Literature Review Guidelines (Not only for Social Work!)
- UW-Madison Writing Center Learn How to Write a Review of Literature
- University of Toronto The Literature Review: A Few Tips on Conducting It
- Mindmap The Literature Review in Under 5 Minutes
- << Previous: Find Articles
- Next: Evaluate Sources >>
- Last Updated: Dec 21, 2022 2:03 PM
- URL: https://libguides.ecu.edu/Physics_Astronomy
University Libraries University of Nevada, Reno
- Skill Guides
- Subject Guides
Physics: Systematic Reviews
- Articles/Research Databases
- Selected Web Resources
- U.S. National Laboratories
- Systematic Reviews
What Is a Systematic Review?
Regular literature reviews are simply summaries of the literature on a particular topic. A systematic review, however, is a comprehensive literature review conducted to answer a specific research question. Authors of a systematic review aim to find, code, appraise, and synthesize all of the previous research on their question in an unbiased and well-documented manner. The Preferred Reporting Items for Systematic Reviews and Meta-Analyses (PRISMA) outline the minimum amount of information that needs to be reported at the conclusion of a systematic review project.
Other types of what are known as "evidence syntheses," such as scoping, rapid, and integrative reviews, have varying methodologies. While systematic reviews originated with and continue to be a popular publication type in medicine and other health sciences fields, more and more researchers in other disciplines are choosing to conduct evidence syntheses.
This guide will walk you through the major steps of a systematic review and point you to key resources including Covidence, a systematic review project management tool. For help with systematic reviews and other major literature review projects, please send us an email at [email protected] .
Getting Help with Reviews
Organization such as the Institute of Medicine recommend that you consult a librarian when conducting a systematic review. Librarians at the University of Nevada, Reno can help you:
- Understand best practices for conducting systematic reviews and other evidence syntheses in your discipline
- Choose and formulate a research question
- Decide which review type (e.g., systematic, scoping, rapid, etc.) is the best fit for your project
- Determine what to include and where to register a systematic review protocol
- Select search terms and develop a search strategy
- Identify databases and platforms to search
- Find the full text of articles and other sources
- Become familiar with free citation management (e.g., EndNote, Zotero)
- Get access to you and help using Covidence, a systematic review project management tool
Doing a Systematic Review
- Plan - This is the project planning stage. You and your team will need to develop a good research question, determine the type of review you will conduct (systematic, scoping, rapid, etc.), and establish the inclusion and exclusion criteria (e.g., you're only going to look at studies that use a certain methodology). All of this information needs to be included in your protocol. You'll also need to ensure that the project is viable - has someone already done a systematic review on this topic? Do some searches and check the various protocol registries to find out.
- Identify - Next, a comprehensive search of the literature is undertaken to ensure all studies that meet the predetermined criteria are identified. Each research question is different, so the number and types of databases you'll search - as well as other online publication venues - will vary. Some standards and guidelines specify that certain databases (e.g., MEDLINE, EMBASE) should be searched regardless. Your subject librarian can help you select appropriate databases to search and develop search strings for each of those databases.
- Evaluate - In this step, retrieved articles are screened and sorted using the predetermined inclusion and exclusion criteria. The risk of bias for each included study is also assessed around this time. It's best if you import search results into a citation management tool (see below) to clean up the citations and remove any duplicates. You can then use a tool like Rayyan (see below) to screen the results. You should begin by screening titles and abstracts only, and then you'll examine the full text of any remaining articles. Each study should be reviewed by a minimum of two people on the project team.
- Collect - Each included study is coded and the quantitative or qualitative data contained in these studies is then synthesized. You'll have to either find or develop a coding strategy or form that meets your needs.
- Explain - The synthesized results are articulated and contextualized. What do the results mean? How have they answered your research question?
- Summarize - The final report provides a complete description of the methods and results in a clear, transparent fashion.
Adapted from
Types of reviews, systematic review.
These types of studies employ a systematic method to analyze and synthesize the results of numerous studies. "Systematic" in this case means following a strict set of steps - as outlined by entities like PRISMA and the Institute of Medicine - so as to make the review more reproducible and less biased. Consistent, thorough documentation is also key. Reviews of this type are not meant to be conducted by an individual but rather a (small) team of researchers. Systematic reviews are widely used in the health sciences, often to find a generalized conclusion from multiple evidence-based studies.
Meta-Analysis
A systematic method that uses statistics to analyze the data from numerous studies. The researchers combine the data from studies with similar data types and analyze them as a single, expanded dataset. Meta-analyses are a type of systematic review.
Scoping Review
A scoping review employs the systematic review methodology to explore a broader topic or question rather than a specific and answerable one, as is generally the case with a systematic review. Authors of these types of reviews seek to collect and categorize the existing literature so as to identify any gaps.
Rapid Review
Rapid reviews are systematic reviews conducted under a time constraint. Researchers make use of workarounds to complete the review quickly (e.g., only looking at English-language publications), which can lead to a less thorough and more biased review.
Narrative Review
A traditional literature review that summarizes and synthesizes the findings of numerous original research articles. The purpose and scope of narrative literature reviews vary widely and do not follow a set protocol. Most literature reviews are narrative reviews.
Umbrella Review
Umbrella reviews are, essentially, systematic reviews of systematic reviews. These compile evidence from multiple review studies into one usable document.
Grant, Maria J., and Andrew Booth. “A Typology of Reviews: An Analysis of 14 Review Types and Associated Methodologies.” Health Information & Libraries Journal , vol. 26, no. 2, 2009, pp. 91-108. doi: 10.1111/j.1471-1842.2009.00848.x .
- << Previous: U.S. National Laboratories
Thank you for visiting nature.com. You are using a browser version with limited support for CSS. To obtain the best experience, we recommend you use a more up to date browser (or turn off compatibility mode in Internet Explorer). In the meantime, to ensure continued support, we are displaying the site without styles and JavaScript.
- View all journals
- Explore content
- About the journal
- Publish with us
- Sign up for alerts
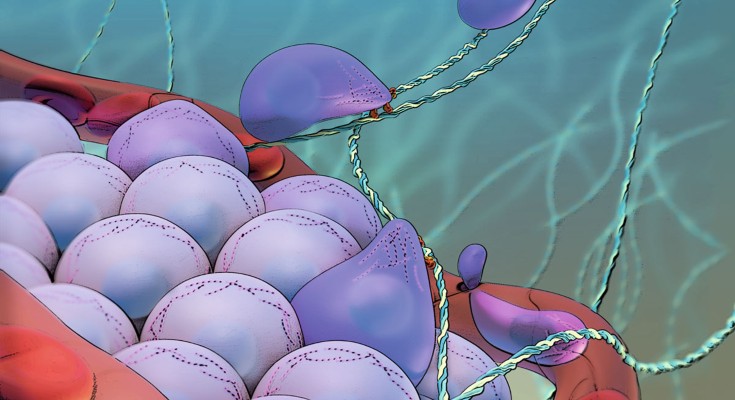
Mechanical properties of human tumour tissues
A Review in this issue discusses the differences in several mechanical proprties of cells and issues that play a role in human cancer developement.
Current issue
Physics words with surprising origins, evolving critical oscillators for hearing.
- A. J. Hudspeth
- Pascal Martin
Food oral processing as a multiscale soft matter physics problem
- Thomas A. Vilgis
Photophysical properties of materials for high-speed photodetection
- Amin Morteza Najarian
- Maral Vafaie
- Edward H. Sargent
Controlling water waves with artificial structures
- Huanyang Chen
How multiscale curvature couples forces to cellular functions
- Marine Luciano
- Caterina Tomba
- Sylvain Gabriele
Announcements
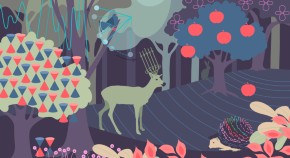
Physics and sustainability
This ongoing collection brings together articles from Nature Reviews journals about how physicists can contribute to environmental sustainability – both by working on questions that have direct relevance to sustainability goals and understanding Earth’s climate, but also by changing the ways physicists work.
Advertisement
Latest Reviews & Analysis
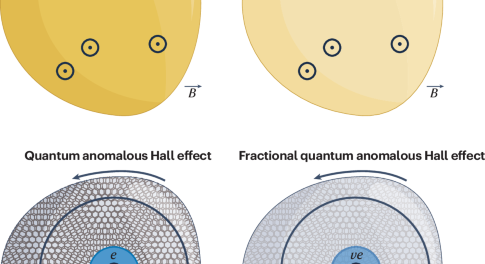
Fractionalized electrons in moiré materials
In the 1980s, the discovery of electron states that fractionalize in the presence of a time-reversal symmetry breaking magnetic field opened up new directions in condensed matter physics. In 2023, evidence has accumulated that a version of these states in which the time-reversal symmetry breaking is spontaneous appears in moiré materials.
- Nicolás Morales-Durán
- Jingtian Shi
- A. H. MacDonald
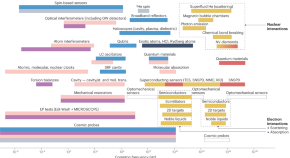
Quantum sensing for particle physics
Quantum sensing exploits properties of quantum systems to go beyond what is possible with traditional measurement techniques, hence opening exciting opportunities in both low-energy and high-energy particle physics experiments.
- Steven D. Bass
- Michael Doser
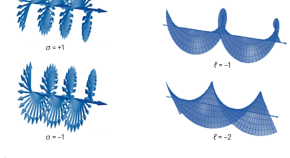
Orbital angular momentum lasers
This Review covers the intriguing physics behind orbital angular momentum lasers, summarizing the exciting prospects at the interface between structured light and structured matter.
- Andrew Forbes
- Light Mkhumbuza
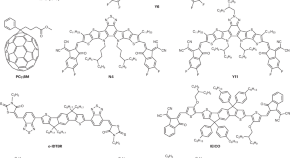
Physical insights into non-fullerene organic photovoltaics
Non-fullerene acceptors have boosted the development of organic photovoltaics. This Review highlights the photophysics and device physics of non-fullerene organic photovoltaics, including exciton generation, diffusion, transport, separation and charge recombination.
- Xiaowei Zhan
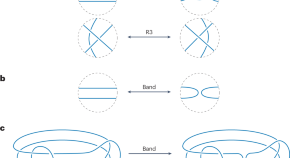
Rigor with machine learning from field theory to the Poincaré conjecture
Machine learning techniques may appear ill-suited for application in fields that prioritize rigor and deep understanding; however, they have recently found unexpected uses in theoretical physics and pure mathematics. In this Perspective, Gukov, Halverson and Ruehle have discussed rigorous applications of machine learning to theoretical physics and pure mathematics.
- Sergei Gukov
- James Halverson
- Fabian Ruehle
Neural operators for accelerating scientific simulations and design
- Kamyar Azizzadenesheli
- Nikola Kovachki
- Anima Anandkumar
Mechanical properties of human tumour tissues and their implications for cancer development
- Andrew Massey
- Jamie Stewart
- Alexander X. Cartagena-Rivera
Anomalies in particle physics and their implications for physics beyond the standard model
- Andreas Crivellin
- Bruce Mellado
News & Comment
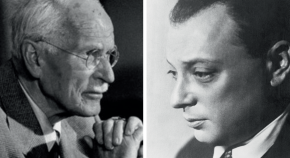
How the unlikely friendship of Pauli and Jung led to the discovery of CPT symmetry
In 1931, the psychoanalyst Carl Jung took on an unusual patient, the brilliant young physicist, Wolfgang Pauli. Arthur I. Miller tells the story of their friendship, how they impacted each other’s work, and reflects on creativity.
- Arthur I. Miller
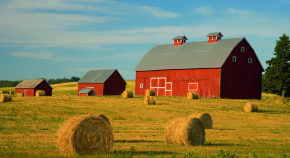
Many everyday English words have a double meaning, being used as physics jargon. This month, we share some of our favourite stories of how physics terms came to be.
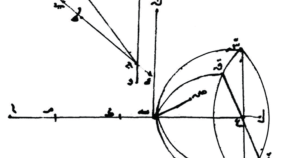
Starting a conversation about social issues in optics and photonics
Science and society are inextricably entangled, but the discussion of social issues in optics and photonics is, at best, treated as peripheral to the field. A group of researchers, technicians, administrative staff, and clinical liaisons share how they came together to start a conversation recognizing these oft-disregarded issues.
- Kimberli Bell
- Taylor M. Cannon
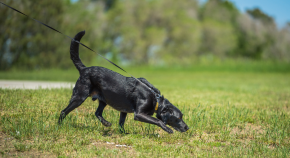
Olfactory cues and memories in animal navigation
Thierry Emonet and Massimo Vergassola discuss what research shows about how animals perform the feat of navigating by smell.
- Thierry Emonet
- Massimo Vergassola
AI is no substitute for having something to say
Good writing is about having something interesting and original to say. Generative AI tools might provide technical help, but they are no substitute for your unique perspective.
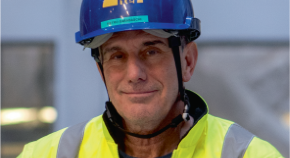
The importance of documenting failure
Pietro Barabaschi, Director General of ITER, calls for measures and incentives to carefully document the entire research process, including dead ends and failures, instead of reporting just the successful final results.
- Pietro Barabaschi
Collections
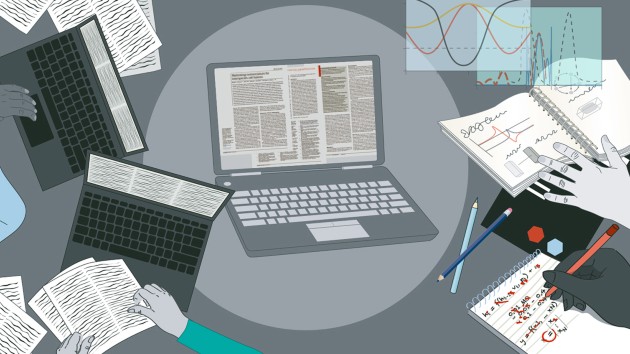
Writing for Nature Reviews Physics
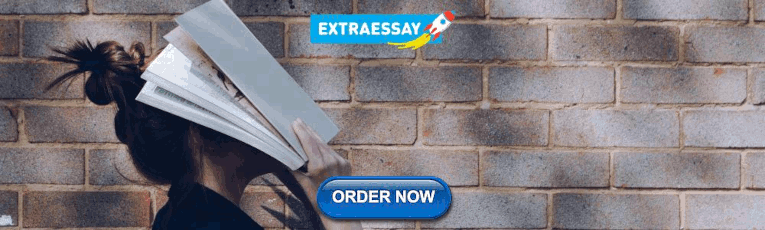
Trending - Altmetric
Lessons from arXiv’s 30 years of information sharing
Stochastic weather and climate models
Noncommuting conserved charges in quantum thermodynamics and beyond
Quick links
- Explore articles by subject
- Guide to authors
- Editorial policies

Physical Review Physics Education Research
- Collections
- Editorial Team
- Open Access
Effect of integrating physics education technology simulations on students’ conceptual understanding in physics: A review of literature
Herbert james banda and joseph nzabahimana, phys. rev. phys. educ. res. 17 , 023108 – published 21 december 2021.
- Citing Articles (21)
- INTRODUCTION
- METHODOLOGY
- RESULTS AND DISCUSSION
- ACKNOWLEDGMENTS
This paper describes a comprehensive review of 31 quasi or experimental research studies from the past decade on the effect of PhET simulations on students’ conceptual understanding of physics. Two questions guided the review: (i) To what extent do PhET simulations enhance students’ conceptual understanding of physics? (ii) What are the best ways to use PhET simulations to enhance conceptual understanding of physics? The reviewed literature provides robust evidence that PhET simulations can significantly enhance students’ conceptual understanding of physics and can be integrated into many active learning instructional environments. The paper also points out gaps and directions of future research and suggests that educators integrate PhET simulations in physics to create more meaningful learning.
- Received 30 June 2021
- Accepted 20 October 2021
DOI: https://doi.org/10.1103/PhysRevPhysEducRes.17.023108

Published by the American Physical Society under the terms of the Creative Commons Attribution 4.0 International license. Further distribution of this work must maintain attribution to the author(s) and the published article’s title, journal citation, and DOI.
Published by the American Physical Society
Physics Subject Headings (PhySH)
- Research Areas
Authors & Affiliations
- 1 African Centre of Excellence for Innovative Teaching and Learning Mathematics and Science (ACEITLMS), University of Rwanda-College of Education (UR-CE), Rukara campus P.O Box 55, Rwamagana, Kayonza, Rwanda
- 2 University of Rwanda-College of Education (UR-CE), Rukara campus P.O Box 55, Rwamagana, Kayonza, Rwanda
- * [email protected]
Article Text
Vol. 17, Iss. 2 — July - December 2021
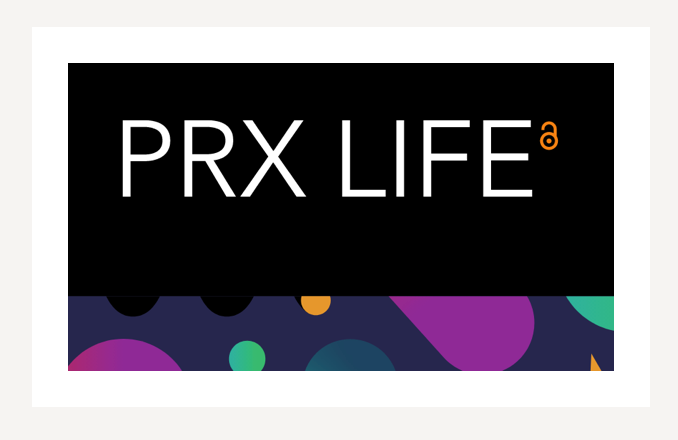
Authorization Required
Other options.
- Buy Article »
- Find an Institution with the Article »
Download & Share
Preferred reporting items for systematic reviews and meta-analysis (PRISMA; [ 14 ]) flow diagram.
Sign up to receive regular email alerts from Physical Review Physics Education Research
Reuse & Permissions
It is not necessary to obtain permission to reuse this article or its components as it is available under the terms of the Creative Commons Attribution 4.0 International license. This license permits unrestricted use, distribution, and reproduction in any medium, provided attribution to the author(s) and the published article's title, journal citation, and DOI are maintained. Please note that some figures may have been included with permission from other third parties. It is your responsibility to obtain the proper permission from the rights holder directly for these figures.
- Forgot your username/password?
- Create an account
Article Lookup
Paste a citation or doi, enter a citation.
- Search Menu
- Volume 2024, Issue 4, April 2024 (In Progress)
- Volume 2024, Issue 3, March 2024
- Advance articles
- Browse content in A General and Mathematical Physics
- Browse content in A0 General physics
- A00 Classical mechanics
- A01 Electromagentism
- A02 Other topics in general physics
- Browse content in A1 Mathematical physics
- A10 Integrable systems and exact solutions
- A11 Solitons
- A12 Rigorous results
- A13 Other topics in mathematical physics
- Browse content in A2 Computational physics
- A20 The algorithm of numerical calculations
- A22 Monte-Carlo simulations
- A23 Molecular dynamics simulations
- A24 Other numerical methods
- Browse content in A3 Nonlinear dynamics
- A30 Dynamical systems (conservative systems)
- A31 The other dynamical systems such as cellular-automata and coupled map lattices
- A32 Quantum chaos
- A33 Classical chaos
- A34 Other topics in nonlinear dynamics
- Browse content in A4 Statistical mechanics - equilibrium systems
- A40 Critical phenomena, phase diagrams, phase transitions
- A41 Spin-glass, random spins
- A42 Classical spins
- A43 Quantum spins
- A44 Neural networks
- A45 Informational statistical physics
- A46 Quantum statistical mechanics
- A47 Other topics in equilibrium statistical mechanics
- Browse content in A5 Statistical mechanics - nonequilibrium systems
- A50 Stochastic processes, stochastic models and percolations
- A51 Relaxations, hysteresis, response, transport in classical systems
- A52 Transport of quantum systems
- A53 Reaction-diffusion systems
- A54 Pattern formation, fracture, self-organizations
- A55 Synchronization; coupled oscillators
- A56 Nonlinear and nonequilibrium phenomena
- A57 Nonequilibrium steady states
- A58 Other topics in nonequilibrium statistical mechanics
- Browse content in A6 Quantum physics and quantum information
- A60 Foundation of quantum mechanics (quantization, geometric phase, entanglement, quantum measurement, locality, contextuality etc)
- A61 Quantum information (quantum computation, quantum cryptography, quantum communication etc)
- A62 Quantum phase transition
- A63 Quantum many-body systems
- A64 Other topics in quantum mechanics
- Browse content in A7 Thermodynamics and thermodynamic processes
- A70 Mathematical theory of thermodynamics
- A73 Other thermal processes
- Browse content in B Theoretical Particle Physics
- Browse content in B0 Gauge field theories
- B00 Gauge theory in general
- B01 Lattice gauge field theories
- B02 Spontaneous symmetry breaking
- B03 Confinement
- B04 Chern Simons theories
- B05 Quantization and formalism
- B06 Other topics in gauge field theories
- Browse content in B1 Supersymmetry
- B10 Extended supersymmetry
- B11 Supergravity
- B12 Supersymmetry breaking
- B13 Supersymmetry phenomenology
- B14 Dynamics of supersymmteric gauge theories
- B15 Supersymmetric quantum mechanics
- B16 Supersymmetric field theory
- B17 Other topics in supersymmetry
- Browse content in B2 String theory
- B20 String duality
- B21 AdS/CFT correspondence
- B22 Black holes in string theory
- B23 Brane and its dynamics
- B24 CFT approach in string theory
- B25 M theory, matrix theory
- B26 Tachyon condensation
- B27 Topological field theory
- B28 String field theory
- B29 Other topics in string theory
- Browse content in B3 Quantum field theory
- B30 General
- B31 Symmetries and anomalies
- B32 Renormalization and renormalization group equation
- B33 Field theories in higher dimensions
- B34 Field theories in lower dimensions
- B35 Solitons, monopoles and instantons, 1/N expansion
- B36 Composite states and effective theories
- B37 Various models of field theory
- B38 Lattice field theories
- B39 Quantization and formalism
- Browse content in B4 Model building
- B40 Beyond the Standard Model
- B41 Compactification and string(-inspired) models
- B42 Grand unified theories
- B43 Models with extra dimensions
- B44 Technicolor and composite models
- B46 Other topics in model building
- Browse content in B5 Weak interactions and related phenomena
- B50 Electromagnetic processes and properties
- B51 B, D, K physics
- B52 CP violation
- B53 Higgs physics
- B54 Neutrino physics
- B55 Quark masses and Standard Model parameters
- B56 Rare decays
- B57 Standard Model
- B58 Supersymmetric Standard Model
- B59 Other topics in weak interactions and related phenomena
- Browse content in B6 Strong interactions and related phenomena
- B60 Chiral lagrangians
- B61 Deep inelastic scattering
- B62 Hadronic colliders
- B64 Lattice QCD
- B65 Perturbative QCD
- B66 Spin and polarization effects
- B67 Sum rules
- B68 Holographic approach to QCD
- B69 Other topics in strong interactions and related phenomena
- Browse content in B7 Astroparticle physics
- B70 Baryogenesis
- B71 Dark matter
- B72 Inflation
- B73 Cosmology of theories beyond the Standard Model
- B74 High energy cosmic rays
- B75 Solar and atmospheric neutrinos
- B77 Other topics in astroparticle physics
- Browse content in B8 Mathematical methods
- B80 Differential and algebraic geometry
- B81 Integrable systems
- B82 Noncommutative geometry
- B83 Matrix models
- B84 Quantum groups
- B85 Bethe ansatz, exact S-matrix
- B86 Statistical methods, random systems
- B87 Other topics in mathematical methods
- Browse content in C Experimental Particle Physics
- Browse content in C0 Standard Model and related topics
- C00 Quantum chromodynamics
- C01 Electroweak model, Higgs bosons, electroweak symmetry breaking
- C02 Cabibbo-Kobayashi-Maskawa quark-mixing matrix
- C03 CP violation
- C04 Neutrino masses, mixing, and oscillations
- C05 Quark model
- C07 Particle properties
- C08 Tests of conservation laws
- C09 Other topics
- Browse content in C1 Hypothetical particles and concepts
- C10 Supersymmmetry
- C12 Grand unified theories
- C14 Extra dimensions
- C18 Heavy vector bosons(W',Z'), leptoquarks, etc
- C19 Other topics
- Browse content in C2 Collider experiments
- C20 Hadron collider experiments
- C21 Lepton collider experiments
- C22 Electron-proton collider experiments
- C23 Other topics
- Browse content in C3 Experiments using particle beams
- C30 Experiments using hadron beams
- C31 Experiments using charged lepton beams
- C32 Experiments using neutrino beams
- C33 Experiments using photon beams
- C34 Other topics
- Browse content in C4 Non-accelerator experiments
- C40 Experiments using RI source
- C41 Laser experiments
- C42 Reactor experiments
- C43 Underground experiments
- C44 Other topics
- Browse content in C5 Other topics in experimental particle physics
- C50 Other topics in experimental particle physics
- Browse content in D Nuclear Physics
- Browse content in D0 Fundamental interactions and nuclear properties
- D00 Nuclear forces (including two nucleon problems)
- D02 Weak interactions in nuclear system (including neutrino-nuclear interactions)
- D03 Electromagnetic interactions in nuclear system
- D04 Nuclear matter and bulk properties of nuclei
- D05 Few-body problems in nuclear system
- D06 Effective interactions in nuclear system
- Browse content in D1 Nuclear structure
- D10 Nuclear many-body theories
- D11 Models of nuclear structure
- D12 General properties of nuclei --- systematics and theoretical analysis
- D13 Stable and unstable nuclei
- D14 Hypernuclei
- D15 Mesic nuclei and exotic atoms
- Browse content in D2 Nuclear reactions and decays
- D20 General reaction theories
- D21 Models of nuclear reactions
- D22 Light ion reactions (A<=4)
- D23 Heavy-ion reactions (low and intermediate energies)
- D24 Photon and lepton reactions
- D25 Hadron reactions
- D26 Fusion, fusion-fission reactions and superheavy nuclei
- D27 Reactions induced by unstable nuclei
- D28 Relativistic heavy-ion collisions
- D29 Nuclear decays and radioactivities (including fission)
- Browse content in D3 Quarks, hadrons and QCD in nuclear physics
- D30 Quark matter
- D31 Quark-gluon plasma
- D32 Hadron structure and interactions
- D33 Hadrons and quarks in nuclear matter
- D34 Lattice QCD calculations in nuclear physics
- Browse content in D4 Nuclear astrophysics
- D40 Nucleosynthesis
- D41 Nuclear matter aspects in nuclear astrophysics
- D42 Nuclear physics aspects in explosive environments
- Browse content in D5 Other topics in nuclear physics
- D50 Other topics in nuclear physics
- Browse content in E Theoretical Astrophysics and Cosmology
- Browse content in E0 Gravity
- E00 Gravity in general
- E01 Relativity
- E02 Gravitational waves
- E03 Alternative theory of gravity
- E04 Higher-dimensional theories
- E05 Quantum gravity
- Browse content in E1 Basic astrophysical processes
- E10 Astrophysical processes in general
- E11 Radiative processes and thermodynamics
- E12 Chemical and nuclear reactions
- E13 Kinetic theory and plasma
- E14 Hydrodynamics and magnetohydrodynamics
- E15 Relativistic dynamics
- Browse content in E2 Stars and stellar systems
- E20 Stars and stellar systems in general
- E21 The sun and solar system
- E23 Interstellar matter and magnetic fields
- E24 Star formation
- E25 Stellar structure and evolution
- E26 Supernovae
- E27 Galaxies and clusters
- E28 Extragalactic medium and fields
- Browse content in E3 Compact objects ?
- E30 Compact objects in general
- E31 Black holes
- E32 Neutron stars
- E33 Pulsars
- E34 Accretion, accretion disks
- E35 Relativistic jets
- E36 Massive black holes
- E37 Gamma ray bursts
- E38 Physics of strong fields
- Browse content in E4 Cosmic rays and neutrinos
- E41 Cosmic rays
- E42 Acceleration of particles
- E43 Propagation of cosmic rays
- E44 Cosmic gamma rays
- E45 Neutrinos
- Browse content in E5 Large scale structure of the universe
- E50 Large scale structure in general
- E51 Superclusters and voids
- E52 Statistical analysis of large scale structure
- E53 Large scale structure formation
- E55 Cosmological simulations
- E56 Cosmological perturbation theory
- Browse content in E6 Observational cosmology
- E60 Observational cosmology in general
- E61 Cosmometry
- E62 Gravitational lensing
- E63 Cosmic background radiations
- E64 Dark energy and dark matter
- E65 Probes of cosmology
- Browse content in E7 Particle cosmology
- E70 Particle cosmology in general
- E72 Baryon asymmetry
- E73 Cosmological phase transitions and topological defects
- E74 Cosmology of physics beyond the Standard Model
- E75 Physics of the early universe
- E76 Quantum field theory on curved space
- Browse content in E8 Inflation and cosmogenesis
- E80 Inflation and cosmology in general
- E81 Inflation
- E83 String theory and cosmology
- E84 Extra dimensions
- E86 Quantum cosmology
- Browse content in F Experimental Astrophysics
- Browse content in F0 Cosmic ray particles
- F00 Instrumentation and technique
- F02 Origin, composition, propagation and interactions of cosmic rays
- F03 Ultra-high energy phenomena of cosmic rays
- F04 Other topics
- Browse content in F1 Photons
- F10 Instrumentation and technique
- F11 Radiation from galactic objects
- F12 Radiation from extragalactic objects
- F14 Cosmic microwave background and extragalactic background lights
- F15 Other topics
- Browse content in F2 Neutrino
- F20 Instrumentation and technique
- F21 Solar, atmospheric and earth-originated neutrinos
- F22 Neutrinos from supernova remnant and other astronomical objects
- F23 Neutrino mass, , mixing, oscillation and interaction
- Browse content in F3 Gravitational wave
- F30 Instrumentation and technique
- F31 Expectation and estimation of gravitational radiation
- F32 Calibration and operation of gravitational wave detector
- F33 Network system, coincident signal in other radiation bands
- F34 Other topics
- Browse content in F4 Dark matter, dark energy and particle physics
- F40 Instrumentation and technique
- F41 Laboratory experiments
- F43 Interpretation and explanation of observation and experiment
- Browse content in G Beam Physics
- Browse content in G0 Accelerators
- G00 Colliders
- G02 Ion accelerators
- G03 Electron accelerators
- G04 Beam sources
- G05 Accelerator components
- G06 Accelerator design
- Browse content in G1 Physics of beams
- G10 Beam dynamics
- G11 Beam instabilities and cures
- G12 Beam measurement and manipulation
- G13 Interaction of beams
- G15 Accelerator theory
- Browse content in G2 Application of beams
- G20 Scientific application
- G22 Industrial application
- Browse content in H Instrumentations and Technologies for Physics
- Browse content in H0 General issue for instrumentation
- H01 Concepts of the detector
- H02 Simulation and detector modeling
- H04 Dosimetry and apparatus
- Browse content in H1 Detectors, apparatus and methods for the physics using accelerators
- H10 Experimental detector systems
- H11 Gaseous detectors
- H12 Semiconductor detectors
- H13 Calorimeters
- H14 Particle identification
- H15 Photon detectors
- H16 Neutrino detectors
- Browse content in H2 Detectors, apparatus and methods for non-accelerator physics
- H20 Instrumentation for underground experiments
- H21 Instrumentation for ground observatory
- H22 Instrumentation for space observatory
- Browse content in H3 Detector readout concepts, electronics, trigger and data acquisition methods
- H33 Digital Signal Processor (DSP) and Field-Programmable Gate Array (FPGA)
- H34 Data acquisition
- Browse content in H4 Software and analysis related issue for instrumentation
- H40 Computing, data processing, data reduction methods
- H41 Image processing
- H42 Pattern recognition, cluster finding, calibration and fitting methods
- H43 Software architectures
- Browse content in H5 Engineering and technical issues
- H50 Detector system design, construction technologies and materials
- H51 Manufacturing
- H54 Gas systems and purification
- Browse content in I Condensed Matter Physics
- Browse content in I0 Structure, mechanical and acoustical properties
- I00 Structure of liquids and solids
- I01 Equations of state
- I02 Phase equilibria and phase transitions
- I04 Mechanical properties
- I07 Acoustical properties
- I08 Other topics
- Browse content in I1 Thermal properties and nonelectronic transport properties
- I10 Thermal properties
- I11 Thermal transport
- I12 Ionic transport
- I14 Other topics
- Browse content in I2 Quantum fluids and solids
- I20 Liquid and solid helium
- I22 Quantum states of cold gases
- I23 Other topics
- Browse content in I3 Low dimensional systems -nonelectronic properties
- I30 Surfaces, interfaces and thin films
- I32 Graphene, fullerene
- I36 Other topics
- Browse content in I4 Electron states in condensed matter
- I42 Organics
- I43 d- and f- electron systems
- I44 First-principles calculations
- I45 Mott transitions
- I46 Strong correlations
- I47 Other topics
- Browse content in I5 Electronic transport properties
- I50 Disordered systems, Anderson transitions
- I51 Hall effect
- I52 Magnetoresistance
- I53 Thermal transport
- I55 Spin transport
- I56 Other topics
- Browse content in I6 Superconductivity
- I60 Mechanism and paring symmetry
- I61 Phenomenology
- I62 Vortices
- I63 Tunnel junction and Josephson effect
- I64 High-Tc superconductors and related materials
- I67 Light-element superconductors
- I68 Other topics
- Browse content in I7 Magnetic and dielectric properties
- I70 Magnetic transitions
- I71 Frustration
- I73 Magnetic resonance
- I74 Magnetism in nanosystems
- I75 Spintronics
- I76 Dielectric properties
- I77 Orbital effects
- I78 Multiferroics
- I79 Other topics
- Browse content in I8 Optical properties
- I81 Nonlinear optics
- I84 Ultrafast phenomena
- I85 Other topics
- Browse content in I9 Low dimensional systems -electronic properties
- I90 Surfaces, interfaces and thin films
- I92 Graphene, fullerene
- I94 Quantum dot
- I95 Other nanostructures
- I96 Quantum Hall effect
- I97 Other topics
- Browse content in J Cross-Disciplinary Physics
- Browse content in J0 Mechanics, elasticity and rheology
- J01 Rheology
- J02 Linear and nonlinear elasticity
- Browse content in J1 Fluid dynamics
- J11 Incompressible fluids
- J12 Compressible fluids and dilute gases
- J13 Electro-magnetic fluids
- J14 Fluids in earth physics and astronomy
- J15 Convections and turbulences
- J16 Waves (nonlinear waves, sound waves, shock waves)
- J18 Vortices
- J19 Other topics in fluid dynamics
- Browse content in J2 Plasma physics
- J20 Nuclear fusions
- J21 Plasma astrophysics
- J22 Waves, heating, instabilities
- J24 Nonlinear phenomena (self-organizations, chaos, turbulences)
- J25 High energy, high density plasma, strongly coupled systems
- J27 Magnetic reconnections, particle accelerations, dynamo
- J28 Non-neutral plasma, dust plasma
- J29 Other topics in plasma physics
- Browse content in J3 Chemical physics
- J32 Solutions and liquids
- J33 Quantum chemistry, electronic states
- J34 Photosynthesis, optical response in biology
- J35 Supercooled liquids and glasses
- J36 Other topics in chemical physics
- Browse content in J4 Soft-matter physics
- J40 Liquid crystals
- J41 Polymer physics
- J43 Glassy systems
- J44 Granular physics
- J45 Other topics in soft-matter physics
- Browse content in J5 Biophysics
- J50 Proteins, nucleic acids, biomembranes, bio-supramolecules
- J53 Biomechanics, physics of biomolecules
- J56 Other topics in biophysics
- Browse content in J6 Geophysics
- J61 Geofluid mechanics, sand motion
- J63 Other topics in geophysics
- Browse content in J7 Others
- J70 Traffic flows and pedestrian dynamics
- J71 Econophysics, social physics
- J72 Physics of games and sports
- J73 Environmental physics
- J74 Other topics
- Author Guidelines
- Submission Site
- Subjects Covered
- Publish with us
- About Progress of Theoretical and Experimental Physics
- PTEP 10th Anniversary Video
- About The Physical Society of Japan
- Editorial Board
- Self-Archiving Policy
- On AI-Based Writing Tools
- Journals on Oxford Academic
- Books on Oxford Academic
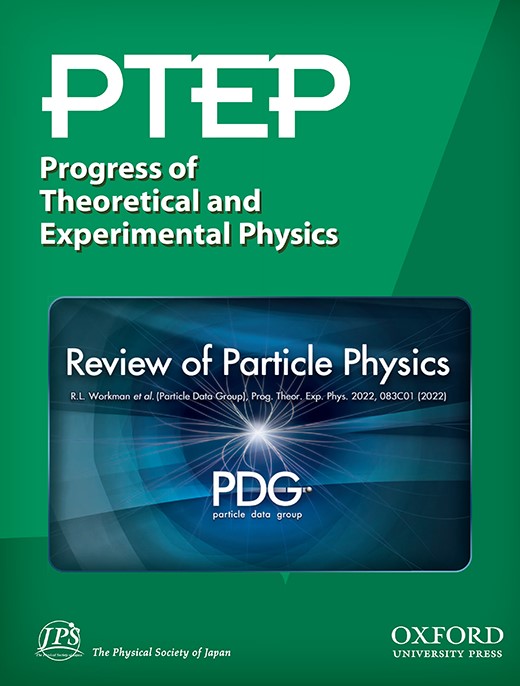
Article Contents
Note to readers, funding statement, review of particle physics.
- Article contents
- Figures & tables
- Supplementary Data
Particle Data Group, R L Workman, V D Burkert, V Crede, E Klempt, U Thoma, L Tiator, K Agashe, G Aielli, B C Allanach, C Amsler, M Antonelli, E C Aschenauer, D M Asner, H Baer, Sw Banerjee, R M Barnett, L Baudis, C W Bauer, J J Beatty, V I Belousov, J Beringer, A Bettini, O Biebel, K M Black, E Blucher, R Bonventre, V V Bryzgalov, O Buchmuller, M A Bychkov, R N Cahn, M Carena, A Ceccucci, A Cerri, R Sekhar Chivukula, G Cowan, K Cranmer, O Cremonesi, G D'Ambrosio, T Damour, D de Florian, A de Gouvêa, T DeGrand, P de Jong, S Demers, B A Dobrescu, M D'Onofrio, M Doser, H K Dreiner, P Eerola, U Egede, S Eidelman, A X El-Khadra, J Ellis, S C Eno, J Erler, V V Ezhela, W Fetscher, B D Fields, A Freitas, H Gallagher, Y Gershtein, T Gherghetta, M C Gonzalez-Garcia, M Goodman, C Grab, A V Gritsan, C Grojean, D E Groom, M Grünewald, A Gurtu, T Gutsche, H E Haber, Matthieu Hamel, C Hanhart, S Hashimoto, Y Hayato, A Hebecker, S Heinemeyer, J J Hernández-Rey, K Hikasa, J Hisano, A Höcker, J Holder, L Hsu, J Huston, T Hyodo, Al Ianni, M Kado, M Karliner, U F Katz, M Kenzie, V A Khoze, S R Klein, F Krauss, M Kreps, P Križan, B Krusche, Y Kwon, O Lahav, J Laiho, L P Lellouch, J Lesgourgues, A R Liddle, Z Ligeti, C-J Lin, C Lippmann, T M Liss, L Littenberg, C Lourenço, K S Lugovsky, S B Lugovsky, A Lusiani, Y Makida, F Maltoni, T Mannel, A V Manohar, W J Marciano, A Masoni, J Matthews, U-G Meißner, I-A Melzer-Pellmann, M Mikhasenko, D J Miller, D Milstead, R E Mitchell, K Mönig, P Molaro, F Moortgat, M Moskovic, K Nakamura, M Narain, P Nason, S Navas, A Nelles, M Neubert, P Nevski, Y Nir, K A Olive, C Patrignani, J A Peacock, V A Petrov, E Pianori, A Pich, A Piepke, F Pietropaolo, A Pomarol, S Pordes, S Profumo, A Quadt, K Rabbertz, J Rademacker, G Raffelt, M Ramsey-Musolf, B N Ratcliff, P Richardson, A Ringwald, D J Robinson, S Roesler, S Rolli, A Romaniouk, L J Rosenberg, J L Rosner, G Rybka, M G Ryskin, R A Ryutin, Y Sakai, S Sarkar, F Sauli, O Schneider, S Schönert, K Scholberg, A J Schwartz, J Schwiening, D Scott, F Sefkow, U Seljak, V Sharma, S R Sharpe, V Shiltsev, G Signorelli, M Silari, F Simon, T Sjöstrand, P Skands, T Skwarnicki, G F Smoot, A Soffer, M S Sozzi, S Spanier, C Spiering, A Stahl, S L Stone, Y Sumino, M J Syphers, F Takahashi, M Tanabashi, J Tanaka, M Taševský, K Terao, K Terashi, J Terning, R S Thorne, M Titov, N P Tkachenko, D R Tovey, K Trabelsi, P Urquijo, G Valencia, R Van de Water, N Varelas, G Venanzoni, L Verde, I Vivarelli, P Vogel, W Vogelsang, V Vorobyev, S P Wakely, W Walkowiak, C W Walter, D Wands, D H Weinberg, E J Weinberg, N Wermes, M White, L R Wiencke, S Willocq, C G Wohl, C L Woody, W-M Yao, M Yokoyama, R Yoshida, G Zanderighi, G P Zeller, O V Zenin, R-Y Zhu, Shi-Lin Zhu, F Zimmermann, P A Zyla, Review of Particle Physics, Progress of Theoretical and Experimental Physics , Volume 2022, Issue 8, August 2022, 083C01, https://doi.org/10.1093/ptep/ptac097
- Permissions Icon Permissions
The Review summarizes much of particle physics and cosmology. Using data from previous editions, plus 2,143 new measurements from 709 papers, we list, evaluate, and average measured properties of gauge bosons and the recently discovered Higgs boson, leptons, quarks, mesons, and baryons. We summarize searches for hypothetical particles such as supersymmetric particles, heavy bosons, axions, dark photons, etc. Particle properties and search limits are listed in Summary Tables. We give numerous tables, figures, formulae, and reviews of topics such as Higgs Boson Physics, Supersymmetry, Grand Unified Theories, Neutrino Mixing, Dark Energy, Dark Matter, Cosmology, Particle Detectors, Colliders, Probability and Statistics. Among the 120 reviews are many that are new or heavily revised, including a new review on Machine Learning, and one on Spectroscopy of Light Meson Resonances.
The Review is divided into two volumes. Volume 1 includes the Summary Tables and 97 review articles. Volume 2 consists of the Particle Listings and contains also 23 reviews that address specific aspects of the data presented in the Listings.
The complete Review (both volumes) is published online on the website of the Particle Data Group ( pdg.lbl.gov ) and in a journal. Volume 1 is available in print as the PDG Book . A Particle Physics Booklet with the Summary Tables and essential tables, figures, and equations from selected review articles is available in print, as a web version optimized for use on phones, and as an Android app.
In the Supplementary Data section below, links for downloading individual sections of the Review have been provided for readers’ convenience.
The 2022 edition of the Review of Particle Physics should be cited as:
R.L. Workman et al. (Particle Data Group), Prog. Theor. Exp. Phys. 2022 , 083C01 (2022)
DOI: 10.1093/ptep/ptac097
For the online version see: https://pdg.lbl.gov/
The publication of the Review of Particle Physics is supported by the Director, Office of Science, Office of High Energy Physics of the U.S. Department of Energy under Contract No. DE–AC02–05CH11231; by an implementing arrangement between the governments of Japan (MEXT: Ministry of Education, Culture, Sports, Science and Technology) and the United States (DOE) on cooperative research and development; by the Italian National Institute of Nuclear Physics (INFN); by the Physical Society of Japan (JPS); and by the European Laboratory for Particle Physics (CERN). Individual collaborators receive support for their PDG activities from their respective institutes or funding agencies.
Supplementary data
Email alerts, astrophysics data system, citing articles via.
- Advertising and Corporate Services
- Journals Career Network
Affiliations
- Online ISSN 2050-3911
- Copyright © 2024 The Physical Society of Japan
- About Oxford Academic
- Publish journals with us
- University press partners
- What we publish
- New features
- Open access
- Institutional account management
- Rights and permissions
- Get help with access
- Accessibility
- Advertising
- Media enquiries
- Oxford University Press
- Oxford Languages
- University of Oxford
Oxford University Press is a department of the University of Oxford. It furthers the University's objective of excellence in research, scholarship, and education by publishing worldwide
- Copyright © 2024 Oxford University Press
- Cookie settings
- Cookie policy
- Privacy policy
- Legal notice
This Feature Is Available To Subscribers Only
Sign In or Create an Account
This PDF is available to Subscribers Only
For full access to this pdf, sign in to an existing account, or purchase an annual subscription.
Help | Advanced Search
Quantum Physics
Title: systematic literature review: quantum machine learning and its applications.
Abstract: Quantum computing is the process of performing calculations using quantum mechanics. This field studies the quantum behavior of certain subatomic particles for subsequent use in performing calculations, as well as for large-scale information processing. These capabilities can give quantum computers an advantage in terms of computational time and cost over classical computers. Nowadays, there are scientific challenges that are impossible to perform by classical computation due to computational complexity or the time the calculation would take, and quantum computation is one of the possible answers. However, current quantum devices have not yet the necessary qubits and are not fault-tolerant enough to achieve these goals. Nonetheless, there are other fields like machine learning or chemistry where quantum computation could be useful with current quantum devices. This manuscript aims to present a Systematic Literature Review of the papers published between 2017 and 2023 to identify, analyze and classify the different algorithms used in quantum machine learning and their applications. Consequently, this study identified 94 articles that used quantum machine learning techniques and algorithms. The main types of found algorithms are quantum implementations of classical machine learning algorithms, such as support vector machines or the k-nearest neighbor model, and classical deep learning algorithms, like quantum neural networks. Many articles try to solve problems currently answered by classical machine learning but using quantum devices and algorithms. Even though results are promising, quantum machine learning is far from achieving its full potential. An improvement in the quantum hardware is required since the existing quantum computers lack enough quality, speed, and scale to allow quantum computing to achieve its full potential.
Submission history
Access paper:.
- HTML (experimental)
- Other Formats

References & Citations
- INSPIRE HEP
- Google Scholar
- Semantic Scholar
BibTeX formatted citation

Bibliographic and Citation Tools
Code, data and media associated with this article, recommenders and search tools.
- Institution
arXivLabs: experimental projects with community collaborators
arXivLabs is a framework that allows collaborators to develop and share new arXiv features directly on our website.
Both individuals and organizations that work with arXivLabs have embraced and accepted our values of openness, community, excellence, and user data privacy. arXiv is committed to these values and only works with partners that adhere to them.
Have an idea for a project that will add value for arXiv's community? Learn more about arXivLabs .
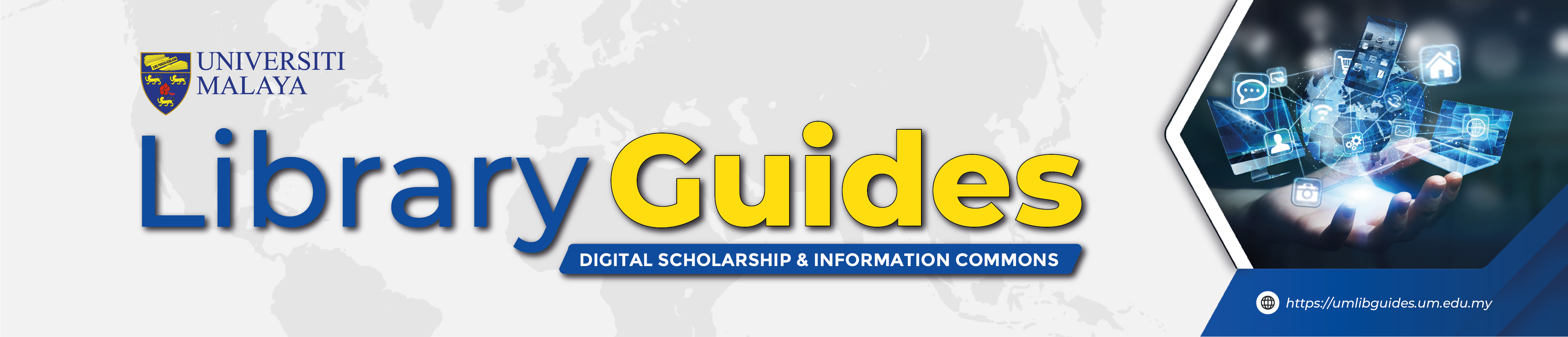
Physics: Literature Review
- Referencing
- Web Resources
- Literature Review
- Associations & Societies
- << Previous: Web Resources
- Next: Books >>
- Last Updated: Sep 21, 2022 10:43 AM
- URL: https://umlibguides.um.edu.my/physics
- Position paper
- Open access
- Published: 28 November 2019
Physics education research for 21 st century learning
- Lei Bao ORCID: orcid.org/0000-0003-3348-4198 1 &
- Kathleen Koenig 2
Disciplinary and Interdisciplinary Science Education Research volume 1 , Article number: 2 ( 2019 ) Cite this article
38k Accesses
71 Citations
2 Altmetric
Metrics details
Education goals have evolved to emphasize student acquisition of the knowledge and attributes necessary to successfully contribute to the workforce and global economy of the twenty-first Century. The new education standards emphasize higher end skills including reasoning, creativity, and open problem solving. Although there is substantial research evidence and consensus around identifying essential twenty-first Century skills, there is a lack of research that focuses on how the related subskills interact and develop over time. This paper provides a brief review of physics education research as a means for providing a context towards future work in promoting deep learning and fostering abilities in high-end reasoning. Through a synthesis of the literature around twenty-first Century skills and physics education, a set of concretely defined education and research goals are suggested for future research, along with how these may impact the next generation physics courses and how physics should be taught in the future.
Introduction
Education is the primary service offered by society to prepare its future generation workforce. The goals of education should therefore meet the demands of the changing world. The concept of learner-centered, active learning has broad, growing support in the research literature as an empirically validated teaching practice that best promotes learning for modern day students (Freeman et al., 2014 ). It stems out of the constructivist view of learning, which emphasizes that it is the learner who needs to actively construct knowledge and the teacher should assume the role of a facilitator rather than the source of knowledge. As implied by the constructivist view, learner-centered education usually emphasizes active-engagement and inquiry style teaching-learning methods, in which the learners can effectively construct their understanding under the guidance of instruction. The learner-centered education also requires educators and researchers to focus their efforts on the learners’ needs, not only to deliver effective teaching-learning approaches, but also to continuously align instructional practices to the education goals of the times. The goals of introductory college courses in science, technology, engineering, and mathematics (STEM) disciplines have constantly evolved from some notion of weed-out courses that emphasize content drilling, to the current constructivist active-engagement type of learning that promotes interest in STEM careers and fosters high-end cognitive abilities.
Following the conceptually defined framework of twenty-first Century teaching and learning, this paper aims to provide contextualized operational definitions of the goals for twenty-first Century learning in physics (and STEM in general) as well as the rationale for the importance of these outcomes for current students. Aligning to the twenty-first Century learning goals, research in physics education is briefly reviewed to provide a context towards future work in promoting deep learning and fostering abilities in high-end reasoning in parallel. Through a synthesis of the literature around twenty-first Century skills and physics education, a set of concretely defined education and research goals are suggested for future research. These goals include: domain-specific research in physics learning; fostering scientific reasoning abilities that are transferable across the STEM disciplines; and dissemination of research-validated curriculum and approaches to teaching and learning. Although this review has a focus on physics education research (PER), it is beneficial to expand the perspective to view physics education in the broader context of STEM learning. Therefore, much of the discussion will blend PER with STEM education as a continuum body of work on teaching and learning.
Education goals for twenty-first century learning
Education goals have evolved to emphasize student acquisition of essential “21 st Century skills”, which define the knowledge and attributes necessary to successfully contribute to the workforce and global economy of the 21st Century (National Research Council, 2011 , 2012a ). In general, these standards seek to transition from emphasizing content-based drilling and memorization towards fostering higher-end skills including reasoning, creativity, and open problem solving (United States Chamber of Commerce, 2017 ). Initiatives on advancing twenty-first Century education focus on skills that converge on three broad clusters: cognitive, interpersonal, and intrapersonal, all of which include a rich set of sub-dimensions.
Within the cognitive domain, multiple competencies have been proposed, including deep learning, non-routine problem solving, systems thinking, critical thinking, computational and information literacy, reasoning and argumentation, and innovation (National Research Council, 2012b ; National Science and Technology Council, 2018 ). Interpersonal skills are those necessary for relating to others, including the ability to work creatively and collaboratively as well as communicate clearly. Intrapersonal skills, on the other hand, reside within the individual and include metacognitive thinking, adaptability, and self-management. These involve the ability to adjust one’s strategy or approach along with the ability to work towards important goals without significant distraction, both essential for sustained success in long-term problem solving and career development.
Although many descriptions exist for what qualifies as twenty-first Century skills, student abilities in scientific reasoning and critical thinking are the most commonly noted and widely studied. They are highly connected with the other cognitive skills of problem solving, decision making, and creative thinking (Bailin, 1996 ; Facione, 1990 ; Fisher, 2001 ; Lipman, 2003 ; Marzano et al., 1988 ), and have been important educational goals since the 1980s (Binkley et al., 2010 ; NCET, 1987 ). As a result, they play a foundational role in defining, assessing, and developing twenty-first Century skills.
The literature for critical thinking is extensive (Bangert-Drowns & Bankert, 1990 ; Facione, 1990 ; Glaser, 1941 ). Various definitions exist with common underlying principles. Broadly defined, critical thinking is the application of the cognitive skills and strategies that aim for and support evidence-based decision making. It is the thinking involved in solving problems, formulating inferences, calculating likelihoods, and making decisions (Halpern, 1999 ). It is the “reasonable reflective thinking focused on deciding what to believe or do” (Ennis, 1993 ). Critical thinking is recognized as a way to understand and evaluate subject matter; producing reliable knowledge and improving thinking itself (Paul, 1990 ; Siegel, 1988 ).
The notion of scientific reasoning is often used to label the set of skills that support critical thinking, problem solving, and creativity in STEM. Broadly defined, scientific reasoning includes the thinking and reasoning skills involved in inquiry, experimentation, evidence evaluation, inference and argument that support the formation and modification of concepts and theories about the natural world; such as the ability to systematically explore a problem, formulate and test hypotheses, manipulate and isolate variables, and observe and evaluate consequences (Bao et al., 2009 ; Zimmerman, 2000 ). Critical thinking and scientific reasoning share many features, where both emphasize evidence-based decision making in multivariable causal conditions. Critical thinking can be promoted through the development of scientific reasoning, which includes student ability to reach a reliable conclusion after identifying a question, formulating hypotheses, gathering relevant data, and logically testing and evaluating the hypothesis. In this way, scientific reasoning can be viewed as a scientific domain instantiation of critical thinking in the context of STEM learning.
In STEM learning, cognitive aspects of the twenty-first Century skills aim to develop reasoning skills, critical thinking skills, and deep understanding, all of which allow students to develop well connected expert-like knowledge structures and engage in meaningful scientific inquiry and problem solving. Within physics education, a core component of STEM education, the learning of conceptual understanding and problem solving remains a current emphasis. However, the fast-changing work environment and technology-driven world require a new set of core knowledge, skills, and habits of mind to solve complex interdisciplinary problems, gather and evaluate evidence, and make sense of information from a variety of sources (Tanenbaum, 2016 ). The education goals in physics are transitioning towards ability fostering as well as extension and integration with other STEM disciplines. Although curriculum that supports these goals is limited, there are a number of attempts, particularly in developing active learning classrooms and inquiry-based laboratory activities, which have demonstrated success. Some of these are described later in this paper as they provide a foundation for future work in physics education.
Interpersonal skills, such as communication and collaboration, are also essential for twenty-first Century problem-solving tasks, which are often open-ended, complex, and team-based. As the world becomes more connected in a multitude of dimensions, tackling significant problems involving complex systems often goes beyond the individual and requires working with others who are increasingly from culturally diverse backgrounds. Due to the rise of communication technologies, being able to articulate thoughts and ideas in a variety of formats and contexts is crucial, as well as the ability to effectively listen or observe to decipher meaning. Interpersonal skills can be promoted by integrating group-learning experiences into the classroom setting, while providing students with the opportunity to engage in open-ended tasks with a team of peer learners who may propose more than one plausible solution. These experiences should be designed such that students must work collaboratively and responsibly in teams to develop creative solutions, which are later disseminated through informative presentations and clearly written scientific reports. Although educational settings in general have moved to providing students with more and more opportunities for collaborative learning, a lack of effective assessments for these important skills has been a limiting factor for producing informative research and widespread implementation. See Liu ( 2010 ) for an overview of measurement instruments reported in the research literature.
Intrapersonal skills are based on the individual and include the ability to manage one’s behavior and emotions to achieve goals. These are especially important for adapting in the fast-evolving collaborative modern work environment and for learning new tasks to solve increasingly challenging interdisciplinary problems, both of which require intellectual openness, work ethic, initiative, and metacognition, to name a few. These skills can be promoted using instruction which, for example, includes metacognitive learning strategies, provides opportunities to make choices and set goals for learning, and explicitly connects to everyday life events. However, like interpersonal skills, the availability of relevant assessments challenges advancement in this area. In this review, the vast amount of studies on interpersonal and intrapersonal skills will not be discussed in order to keep the main focus on the cognitive side of skills and reasoning.
The purpose behind discussing twenty-first Century skills is that this set of skills provides important guidance for establishing essential education goals for modern society and learners. However, although there is substantial research evidence and consensus around identifying necessary twenty-first Century skills, there is a lack of research that focuses on how the related subskills interact and develop over time (Reimers & Chung, 2016 ), with much of the existing research residing in academic literature that is focused on psychology rather than education systems (National Research Council, 2012a ). Therefore, a major and challenging task for discipline-based education researchers and educators is to operationally define discipline-specific goals that align with the twenty-first Century skills for each of the STEM fields. In the following sections, this paper will provide a limited vision of the research endeavors in physics education that can translate the past and current success into sustained impact for twenty-first Century teaching and learning.
Proposed education and research goals
Physics education research (PER) is often considered an early pioneer in discipline-based education research (National Research Council, 2012c ), with well-established, broad, and influential outcomes (e.g., Hake, 1998 ; Hsu, Brewe, Foster, & Harper, 2004 ; McDermott & Redish, 1999 ; Meltzer & Thornton, 2012 ). Through the integration of twenty-first Century skills with the PER literature, a set of broadly defined education and research goals is proposed for future PER work:
Discipline-specific deep learning: Cognitive and education research involving physics learning has established a rich literature on student learning behaviors along with a number of frameworks. Some of the popular frameworks include conceptual understanding and concept change, problem solving, knowledge structure, deep learning, and knowledge integration. Aligned with twenty-first Century skills, future research in physics learning should aim to integrate the multiple areas of existing work, such that they help students develop well integrated knowledge structures in order to achieve deep leaning in physics.
Fostering scientific reasoning for transfer across STEM disciplines: The broad literature in physics learning and scientific reasoning can provide a solid foundation to further develop effective physics education approaches, such as active engagement instruction and inquiry labs, specifically targeting scientific inquiry abilities and reasoning skills. Since scientific reasoning is a more domain-general cognitive ability, success in physics can also more readily inform research and education practices in other STEM fields.
Research, development, assessment, and dissemination of effective education approaches: Developing and maintaining a supportive infrastructure of education research and implementation has always been a challenge, not only in physics but in all STEM areas. The twenty-first Century education requires researchers and instructors across STEM to work together as an extended community in order to construct a sustainable integrated STEM education environment. Through this new infrastructure, effective team-based inquiry learning and meaningful assessment can be delivered to help students develop a comprehensive skills set including deep understanding and scientific reasoning, as well as communication and other non-cognitive abilities.
The suggested research will generate understanding and resources to support education practices that meet the requirements of the Next Generation Science Standards (NGSS), which explicitly emphasize three areas of learning including disciplinary core ideas, crosscutting concepts, and practices (National Research Council, 2012b ). The first goal for promoting deep learning of disciplinary knowledge corresponds well to the NGSS emphasis on disciplinary core ideas, which play a central role in helping students develop well integrated knowledge structures to achieve deep understanding. The second goal on fostering transferable scientific reasoning skills supports the NGSS emphasis on crosscutting concepts and practices. Scientific reasoning skills are crosscutting cognitive abilities that are essential to the development of domain-general concepts and modeling strategies. In addition, the development of scientific reasoning requires inquiry-based learning and practices. Therefore, research on scientific reasoning can produce a valuable knowledge base on education means that are effective for developing crosscutting concepts and promoting meaningful practices in STEM. The third research goal addresses the challenge in the assessment of high-end skills and the dissemination of effective educational approaches, which supports all NGSS initiatives to ensure sustainable development and lasting impact. The following sections will discuss the research literature that provides the foundation for these three research goals and identify the specific challenges that will need to be addressed in future work.
Promoting deep learning in physics education
Physics education for the twenty-first Century aims to foster high-end reasoning skills and promote deep conceptual understanding. However, many traditional education systems place strong emphasis on only problem solving with the expectation that students obtain deep conceptual understanding through repetitive problem-solving practices, which often doesn’t occur (Alonso, 1992 ). This focus on problem solving has been shown to have limitations as a number of studies have revealed disconnections between learning conceptual understanding and problem-solving skills (Chiu, 2001 ; Chiu, Guo, & Treagust, 2007 ; Hoellwarth, Moelter, & Knight, 2005 ; Kim & Pak, 2002 ; Nakhleh, 1993 ; Nakhleh & Mitchell, 1993 ; Nurrenbern & Pickering, 1987 ; Stamovlasis, Tsaparlis, Kamilatos, Papaoikonomou, & Zarotiadou, 2005 ). In fact, drilling in problem solving may actually promote memorization of context-specific solutions with minimal generalization rather than transitioning students from novices to experts.
Towards conceptual understanding and learning, many models and definitions have been established to study and describe student conceptual knowledge states and development. For example, students coming into a physics classroom often hold deeply rooted, stable understandings that differ from expert conceptions. These are commonly referred to as misconceptions or alternative conceptions (Clement, 1982 ; Duit & Treagust, 2003 ; Dykstra Jr, Boyle, & Monarch, 1992 ; Halloun & Hestenes, 1985a , 1985b ). Such students’ conceptions are context dependent and exist as disconnected knowledge fragments, which are strongly situated within specific contexts (Bao & Redish, 2001 , 2006 ; Minstrell, 1992 ).
In modeling students’ knowledge structures, DiSessa’s proposed phenomenological primitives (p-prim) describe a learner’s implicit thinking, cued from specific contexts, as an underpinning cognitive construct for a learner’s expressed conception (DiSessa, 1993 ; Smith III, DiSessa, & Roschelle, 1994 ). Facets, on the other hand, map between the implicit p-prim and concrete statements of beliefs and are developed as discrete and independent units of thought, knowledge, or strategies used by individuals to address specific situations (Minstrell, 1992 ). Ontological categories, defined by Chi, describe student reasoning in the most general sense. Chi believed that these are distinct, stable, and constraining, and that a core reason behind novices’ difficulties in physics is that they think of physics within the category of matter instead of processes (Chi, 1992 ; Chi & Slotta, 1993 ; Chi, Slotta, & De Leeuw, 1994 ; Slotta, Chi, & Joram, 1995 ). More details on conceptual learning and problem solving are well summarized in the literature (Hsu et al., 2004 ; McDermott & Redish, 1999 ), from which a common theme emerges from the models and definitions. That is, learning is context dependent and students with poor conceptual understanding typically have locally connected knowledge structures with isolated conceptual constructs that are unable to establish similarities and contrasts between contexts.
Additionally, this idea of fragmentation is demonstrated through many studies on student problem solving in physics and other fields. It has been shown that a student’s knowledge organization is a key aspect for distinguishing experts from novices (Bagno, Eylon, & Ganiel, 2000 ; Chi, Feltovich, & Glaser, 1981 ; De Jong & Ferguson-Hesler, 1986 ; Eylon & Reif, 1984 ; Ferguson-Hesler & De Jong, 1990 ; Heller & Reif, 1984 ; Larkin, McDermott, Simon, & Simon, 1980 ; Smith, 1992 ; Veldhuis, 1990 ; Wexler, 1982 ). Expert’s knowledge is organized around core principles of physics, which are applied to guide problem solving and develop connections between different domains as well as new, unfamiliar situations (Brown, 1989 ; Perkins & Salomon, 1989 ; Salomon & Perkins, 1989 ). Novices, on the other hand, lack a well-organized knowledge structure and often solve problems by relying on surface features that are directly mapped to certain problem-solving outcomes through memorization (Chi, Bassok, Lewis, Reimann, & Glaser, 1989 ; Hardiman, Dufresne, & Mestre, 1989 ; Schoenfeld & Herrmann, 1982 ).
This lack of organization creates many difficulties in the comprehension of basic concepts and in solving complex problems. This leads to the common complaint that students’ knowledge of physics is reduced to formulas and vague labels of the concepts, which are unable to substantively contribute to meaningful reasoning processes. A novice’s fragmented knowledge structure severely limits the learner’s conceptual understanding. In essence, these students are able to memorize how to approach a problem given specific information but lack the understanding of the underlying concept of the approach, limiting their ability to apply this approach to a novel situation. In order to achieve expert-like understanding, a student’s knowledge structure must integrate all of the fragmented ideas around the core principle to form a coherent and fully connected conceptual framework.
Towards a more general theoretical consideration, students’ alternative conceptions and fragmentation in knowledge structures can be viewed through both the “naïve theory” framework (e.g., Posner, Strike, Hewson, & Gertzog, 1982 ; Vosniadou, Vamvakoussi, & Skopeliti, 2008 ) and the “knowledge in pieces” (DiSessa, 1993 ) perspective. The “naïve theory” framework considers students entering the classroom with stable and coherent ideas (naïve theories) about the natural world that differ from those presented by experts. In the “knowledge in pieces” perspective, student knowledge is constructed in real-time and incorporates context features with the p-prims to form the observed conceptual expressions. Although there exists an ongoing debate between these two views (Kalman & Lattery, 2018 ), it is more productive to focus on their instructional implications for promoting meaningful conceptual change in students’ knowledge structures.
In the process of learning, students may enter the classroom with a range of initial states depending on the population and content. For topics with well-established empirical experiences, students often have developed their own ideas and understanding, while on topics without prior exposure, students may create their initial understanding in real-time based on related prior knowledge and given contextual features (Bao & Redish, 2006 ). These initial states of understanding, regardless of their origin, are usually different from those of experts. Therefore, the main function of teaching and learning is to guide students to modify their initial understanding towards the experts’ views. Although students’ initial understanding may exist as a body of coherent ideas within limited contexts, as students start to change their knowledge structures throughout the learning process, they may evolve into a wide range of transitional states with varying levels of knowledge integration and coherence. The discussion in this brief review on students’ knowledge structures regarding fragmentation and integration are primarily focused on the transitional stages emerged through learning.
The corresponding instructional goal is then to help students more effectively develop an integrated knowledge structure so as to achieve a deep conceptual understanding. From an educator’s perspective, Bloom’s taxonomy of education objectives establishes a hierarchy of six levels of cognitive skills based on their specificity and complexity: Remember (lowest and most specific), Understand, Apply, Analyze, Evaluate, and Create (highest and most general and complex) (Anderson et al., 2001 ; Bloom, Engelhart, Furst, Hill, & Krathwohl, 1956 ). This hierarchy of skills exemplifies the transition of a learner’s cognitive development from a fragmented and contextually situated knowledge structure (novice with low level cognitive skills) to a well-integrated and globally networked expert-like structure (with high level cognitive skills).
As a student’s learning progresses from lower to higher cognitive levels, the student’s knowledge structure becomes more integrated and is easier to transfer across contexts (less context specific). For example, beginning stage students may only be able to memorize and perform limited applications of the features of certain contexts and their conditional variations, with which the students were specifically taught. This leads to the establishment of a locally connected knowledge construct. When a student’s learning progresses from the level of Remember to Understand, the student begins to develop connections among some of the fragmented pieces to form a more fully connected network linking a larger set of contexts, thus advancing into a higher level of understanding. These connections and the ability to transfer between different situations form the basis of deep conceptual understanding. This growth of connections leads to a more complete and integrated cognitive structure, which can be mapped to a higher level on Bloom’s taxonomy. This occurs when students are able to relate a larger number of different contextual and conditional aspects of a concept for analyzing and evaluating to a wider variety of problem situations.
Promoting the growth of connections would appear to aid in student learning. Exactly which teaching methods best facilitate this are dependent on the concepts and skills being learned and should be determined through research. However, it has been well recognized that traditional instruction often fails to help students obtain expert-like conceptual understanding, with many misconceptions still existing after instruction, indicating weak integration within a student’s knowledge structure (McKeachie, 1986 ).
Recognizing the failures of traditional teaching, various research-informed teaching methods have been developed to enhance student conceptual learning along with diagnostic tests, which aim to measure the existence of misconceptions. Most advances in teaching methods focus on the inclusion of inquiry-based interactive-engagement elements in lecture, recitations, and labs. In physics education, these methods were popularized after Hake’s landmark study demonstrated the effectiveness of interactive-engagement over traditional lectures (Hake, 1998 ). Some of these methods include the use of peer instruction (Mazur, 1997 ), personal response systems (e.g., Reay, Bao, Li, Warnakulasooriya, & Baugh, 2005 ), studio-style instruction (Beichner et al., 2007 ), and inquiry-based learning (Etkina & Van Heuvelen, 2001 ; Laws, 2004 ; McDermott, 1996 ; Thornton & Sokoloff, 1998 ). The key approach of these methods aims to improve student learning by carefully targeting deficits in student knowledge and actively encouraging students to explore and discuss. Rather than rote memorization, these approaches help promote generalization and deeper conceptual understanding by building connections between knowledge elements.
Based on the literature, including Bloom’s taxonomy and the new education standards that emphasize twenty-first Century skills, a common focus on teaching and learning can be identified. This focus emphasizes helping students develop connections among fragmented segments of their knowledge pieces and is aligned with the knowledge integration perspective, which focuses on helping students develop and refine their knowledge structure toward a more coherently organized and extensively connected network of ideas (Lee, Liu, & Linn, 2011 ; Linn, 2005 ; Nordine, Krajcik, & Fortus, 2011 ; Shen, Liu, & Chang, 2017 ). For meaningful learning to occur, new concepts must be integrated into a learner’s existing knowledge structure by linking the new knowledge to already understood concepts.
Forming an integrated knowledge structure is therefore essential to achieving deep learning, not only in physics but also in all STEM fields. However, defining what connections must occur at different stages of learning, as well as understanding the instructional methods necessary for effectively developing such connections within each STEM disciplinary context, are necessary for current and future research. Together these will provide the much needed foundational knowledge base to guide the development of the next generation of curriculum and classroom environment designed around twenty-first Century learning.
Developing scientific reasoning with inquiry labs
Scientific reasoning is part of the widely emphasized cognitive strand of twenty-first Century skills. Through development of scientific reasoning skills, students’ critical thinking, open-ended problem-solving abilities, and decision-making skills can be improved. In this way, targeting scientific reasoning as a curricular objective is aligned with the goals emphasized in twenty-first Century education. Also, there is a growing body of research on the importance of student development of scientific reasoning, which have been found to positively correlate with course achievement (Cavallo, Rozman, Blickenstaff, & Walker, 2003 ; Johnson & Lawson, 1998 ), improvement on concept tests (Coletta & Phillips, 2005 ; She & Liao, 2010 ), engagement in higher levels of problem solving (Cracolice, Deming, & Ehlert, 2008 ; Fabby & Koenig, 2013 ); and success on transfer (Ates & Cataloglu, 2007 ; Jensen & Lawson, 2011 ).
Unfortunately, research has shown that college students are lacking in scientific reasoning. Lawson ( 1992 ) found that ~ 50% of intro biology students are not capable of applying scientific reasoning in learning, including the ability to develop hypotheses, control variables, and design experiments; all necessary for meaningful scientific inquiry. Research has also found that traditional courses do not significantly develop these abilities, with pre-to-post-test gains of 1%–2%, while inquiry-based courses have gains around 7% (Koenig, Schen, & Bao, 2012 ; Koenig, Schen, Edwards, & Bao, 2012 ). Others found that undergraduates have difficulty developing evidence-based decisions and differentiating between and linking evidence with claims (Kuhn, 1992 ; Shaw, 1996 ; Zeineddin & Abd-El-Khalick, 2010 ). A large scale international study suggested that learning of physics content knowledge with traditional teaching practices does not improve students’ scientific reasoning skills (Bao et al., 2009 ).
Aligned to twenty-first Century learning, it is important to implement curriculum that is specifically designed for developing scientific reasoning abilities within current education settings. Although traditional lectures may continue for decades due to infrastructure constraints, a unique opportunity can be found in the lab curriculum, which may be more readily transformed to include hands-on minds-on group learning activities that are ideal for developing students’ abilities in scientific inquiry and reasoning.
For well over a century, the laboratory has held a distinctive role in student learning (Meltzer & Otero, 2015 ). However, many existing labs, which haven’t changed much since the late 1980s, have received criticism for their outdated cookbook style that lacks effectiveness in developing high-end skills. In addition, labs have been primarily used as a means for verifying the physical principles presented in lecture, and unfortunately, Hofstein and Lunetta ( 1982 ) found in an early review of the literature that research was unable to demonstrate the impact of the lab on student content learning.
About this same time, a shift towards a constructivist view of learning gained popularity and influenced lab curriculum development towards engaging students in the process of constructing knowledge through science inquiry. Curricula, such as Physics by Inquiry (McDermott, 1996 ), Real-Time Physics (Sokoloff, Thornton, & Laws, 2011 ), and Workshop Physics (Laws, 2004 ), were developed with a primary focus on engaging students in cognitive conflict to address misconceptions. Although these approaches have been shown to be highly successful in improving deep learning of physics concepts (McDermott & Redish, 1999 ), the emphasis on conceptual learning does not sufficiently impact the domain general scientific reasoning skills necessitated in the goals of twenty-first Century learning.
Reform in science education, both in terms of targeted content and skills, along with the emergence of knowledge regarding human cognition and learning (Bransford, Brown, & Cocking, 2000 ), have generated renewed interest in the potential of inquiry-based lab settings for skill development. In these types of hands-on minds-on learning, students apply the methods and procedures of science inquiry to investigate phenomena and construct scientific claims, solve problems, and communicate outcomes, which holds promise for developing both conceptual understanding and scientific reasoning skills in parallel (Trowbridge, Bybee, & Powell, 2000 ). In addition, the availability of technology to enhance inquiry-based learning has seen exponential growth, along with the emergence of more appropriate research methodologies to support research on student learning.
Although inquiry-based labs hold promise for developing students’ high-end reasoning, analytic, and scientific inquiry abilities, these educational endeavors have not become widespread, with many existing physics laboratory courses still viewed merely as a place to illustrate the physical principles from the lecture course (Meltzer & Otero, 2015 ). Developing scientific ideas from practical experiences, however, is a complex process. Students need sufficient time and opportunity for interaction and reflection on complex, investigative tasks. Blended learning, which merges lecture and lab (such as studio style courses), addresses this issue to some extent, but has experienced limited adoption, likely due to the demanding infrastructure resources, including dedicated technology-intensive classroom space, equipment and maintenance costs, and fully committed trained staff.
Therefore, there is an immediate need to transform the existing standalone lab courses, within the constraints of the existing education infrastructure, into more inquiry-based designs, with one of its primary goals dedicated to developing scientific reasoning skills. These labs should center on constructing knowledge, along with hands-on minds-on practical skills and scientific reasoning, to support modeling a problem, designing and implementing experiments, analyzing and interpreting data, drawing and evaluating conclusions, and effective communication. In particular, training on scientific reasoning needs to be explicitly addressed in the lab curriculum, which should contain components specifically targeting a set of operationally-defined scientific reasoning skills, such as ability to control variables or engage in multivariate causal reasoning. Although effective inquiry may also implicitly develop some aspects of scientific reasoning skills, such development is far less efficient and varies with context when the primary focus is on conceptual learning.
Several recent efforts to enhance the standalone lab course have shown promise in supporting education goals that better align with twenty-first Century learning. For example, the Investigative Science Learning Environment (ISLE) labs involve a series of tasks designed to help students develop the “habits of mind” of scientists and engineers (Etkina et al., 2006 ). The curriculum targets reasoning as well as the lab learning outcomes published by the American Association of Physics Teachers (Kozminski et al., 2014 ). Operationally, ISLE methods focus on scaffolding students’ developing conceptual understanding using inquiry learning without a heavy emphasis on cognitive conflict, making it more appropriate and effective for entry level students and K-12 teachers.
Likewise, Koenig, Wood, Bortner, and Bao ( 2019 ) have developed a lab curriculum that is intentionally designed around the twenty-first Century learning goals for developing cognitive, interpersonal, and intrapersonal abilities. In terms of the cognitive domain, the lab learning outcomes center on critical thinking and scientific reasoning but do so through operationally defined sub-skills, all of which are transferrable across STEM. These selected sub-skills are found in the research literature, and include the ability to control variables and engage in data analytics and causal reasoning. For each targeted sub-skill, a series of pre-lab and in-class activities provide students with repeated, deliberate practice within multiple hypothetical science-based scenarios followed by real inquiry-based lab contexts. This explicit instructional strategy has been shown to be essential for the development of scientific reasoning (Chen & Klahr, 1999 ). In addition, the Karplus Learning Cycle (Karplus, 1964 ) provides the foundation for the structure of the lab activities and involves cycles of exploration, concept introduction, and concept application. The curricular framework is such that as the course progresses, the students engage in increasingly complex tasks, which allow students the opportunity to learn gradually through a progression from simple to complex skills.
As part of this same curriculum, students’ interpersonal skills are developed, in part, through teamwork, as students work in groups of 3 or 4 to address open-ended research questions, such as, What impacts the period of a pendulum? In addition, due to time constraints, students learn early on about the importance of working together in an efficient manor towards a common goal, with one set of written lab records per team submitted after each lab. Checkpoints built into all in-class activities involve Socratic dialogue between the instructor and students and promote oral communication. This use of directed questioning guides students in articulating their reasoning behind decisions and claims made, while supporting the development of scientific reasoning and conceptual understanding in parallel (Hake, 1992 ). Students’ intrapersonal skills, as well as communication skills, are promoted through the submission of individual lab reports. These reports require students to reflect upon their learning over each of four multi-week experiments and synthesize their ideas into evidence-based arguments, which support a claim. Due to the length of several weeks over which students collect data for each of these reports, the ability to organize the data and manage their time becomes essential.
Despite the growing emphasis on research and development of curriculum that targets twenty-first Century learning, converting a traditionally taught lab course into a meaningful inquiry-based learning environment is challenging in current reform efforts. Typically, the biggest challenge is a lack of resources; including faculty time to create or adapt inquiry-based materials for the local setting, training faculty and graduate student instructors who are likely unfamiliar with this approach, and the potential cost of new equipment. Koenig et al. ( 2019 ) addressed these potential implementation barriers by designing curriculum with these challenges in mind. That is, the curriculum was designed as a flexible set of modules that target specific sub-skills, with each module consisting of pre-lab (hypothetical) and in-lab (real) activities. Each module was designed around a curricular framework such that an adopting institution can use the materials as written, or can incorporate their existing equipment and experiments into the framework with minimal effort. Other non-traditional approaches have also been experimented with, such as the work by Sobhanzadeh, Kalman, and Thompson ( 2017 ), which targets typical misconceptions by using conceptual questions to engage students in making a prediction, designing and conducting a related experiment, and determining whether or not the results support the hypothesis.
Another challenge for inquiry labs is the assessment of skills-based learning outcomes. For assessment of scientific reasoning, a new instrument on inquiry in scientific thinking analytics and reasoning (iSTAR) has been developed, which can be easily implemented across large numbers of students as both a pre- and post-test to assess gains. iSTAR assesses reasoning skills necessary in the systematical conduct of scientific inquiry, which includes the ability to explore a problem, formulate and test hypotheses, manipulate and isolate variables, and observe and evaluate the consequences (see www.istarassessment.org ). The new instrument expands upon the commonly used classroom test of scientific reasoning (Lawson, 1978 , 2000 ), which has been identified with a number of validity weaknesses and a ceiling effect for college students (Bao, Xiao, Koenig, & Han, 2018 ).
Many education innovations need supporting infrastructures that can ensure adoption and lasting impact. However, making large-scale changes to current education settings can be risky, if not impossible. New education approaches, therefore, need to be designed to adapt to current environmental constraints. Since higher-end skills are a primary focus of twenty-first Century learning, which are most effectively developed in inquiry-based group settings, transforming current lecture and lab courses into this new format is critical. Although this transformation presents great challenges, promising solutions have already emerged from various research efforts. Perhaps the biggest challenge is for STEM educators and researchers to form an alliance to work together to re-engineer many details of the current education infrastructure in order to overcome the multitude of implementation obstacles.
This paper attempts to identify a few central ideas to provide a broad picture for future research and development in physics education, or STEM education in general, to promote twenty-first Century learning. Through a synthesis of the existing literature within the authors’ limited scope, a number of views surface.
Education is a service to prepare (not to select) the future workforce and should be designed as learner-centered, with the education goals and teaching-learning methods tailored to the needs and characteristics of the learners themselves. Given space constraints, the reader is referred to the meta-analysis conducted by Freeman et al. ( 2014 ), which provides strong support for learner-centered instruction. The changing world of the twenty-first Century informs the establishment of new education goals, which should be used to guide research and development of teaching and learning for present day students. Aligned to twenty-first Century learning, the new science standards have set the goals for STEM education to transition towards promoting deep learning of disciplinary knowledge, thereby building upon decades of research in PER, while fostering a wide range of general high-end cognitive and non-cognitive abilities that are transferable across all disciplines.
Following these education goals, more research is needed to operationally define and assess the desired high-end reasoning abilities. Building on a clear definition with effective assessments, a large number of empirical studies are needed to investigate how high-end abilities can be developed in parallel with deep learning of concepts, such that what is learned can be generalized to impact the development of curriculum and teaching methods which promote skills-based learning across all STEM fields. Specifically for PER, future research should emphasize knowledge integration to promote deep conceptual understanding in physics along with inquiry learning to foster scientific reasoning. Integration of physics learning in contexts that connect to other STEM disciplines is also an area for more research. Cross-cutting, interdisciplinary connections are becoming important features of the future generation physics curriculum and defines how physics should be taught collaboratively with other STEM courses.
This paper proposed meaningful areas for future research that are aligned with clearly defined education goals for twenty-first Century learning. Based on the existing literature, a number of challenges are noted for future directions of research, including the need for:
clear and operational definitions of goals to guide research and practice
concrete operational definitions of high-end abilities for which students are expected to develop
effective assessment methods and instruments to measure high-end abilities and other components of twenty-first Century learning
a knowledge base of the curriculum and teaching and learning environments that effectively support the development of advanced skills
integration of knowledge and ability development regarding within-discipline and cross-discipline learning in STEM
effective means to disseminate successful education practices
The list is by no means exhaustive, but these themes emerge above others. In addition, the high-end abilities discussed in this paper focus primarily on scientific reasoning, which is highly connected to other skills, such as critical thinking, systems thinking, multivariable modeling, computational thinking, design thinking, etc. These abilities are expected to develop in STEM learning, although some may be emphasized more within certain disciplines than others. Due to the limited scope of this paper, not all of these abilities were discussed in detail but should be considered an integral part of STEM learning.
Finally, a metacognitive position on education research is worth reflection. One important understanding is that the fundamental learning mechanism hasn’t changed, although the context in which learning occurs has evolved rapidly as a manifestation of the fast-forwarding technology world. Since learning is a process at the interface between a learner’s mind and the environment, the main focus of educators should always be on the learner’s interaction with the environment, not just the environment. In recent education developments, many new learning platforms have emerged at an exponential rate, such as the massive open online courses (MOOCs), STEM creative labs, and other online learning resources, to name a few. As attractive as these may be, it is risky to indiscriminately follow trends in education technology and commercially-incentivized initiatives before such interventions are shown to be effective by research. Trends come and go but educators foster students who have only a limited time to experience education. Therefore, delivering effective education is a high-stakes task and needs to be carefully and ethically planned and implemented. When game-changing opportunities emerge, one needs to not only consider the winners (and what they can win), but also the impact on all that is involved.
Based on a century of education research, consensus has settled on a fundamental mechanism of teaching and learning, which suggests that knowledge is developed within a learner through constructive processes and that team-based guided scientific inquiry is an effective method for promoting deep learning of content knowledge as well as developing high-end cognitive abilities, such as scientific reasoning. Emerging technology and methods should serve to facilitate (not to replace) such learning by providing more effective education settings and conveniently accessible resources. This is an important relationship that should survive many generations of technological and societal changes in the future to come. From a physicist’s point of view, a fundamental relation like this can be considered the “mechanics” of teaching and learning. Therefore, educators and researchers should hold on to these few fundamental principles without being distracted by the surfacing ripples of the world’s motion forward.
Availability of data and materials
Not applicable.
Abbreviations
American Association of Physics Teachers
Investigative Science Learning Environment
Inquiry in Scientific Thinking Analytics and Reasoning
Massive open online course
New Generation Science Standards
- Physics education research
Science Technology Engineering and Math
Alonso, M. (1992). Problem solving vs. conceptual understanding. American Journal of Physics , 60 (9), 777–778. https://doi.org/10.1119/1.17056 .
Article Google Scholar
Anderson, L. W., Krathwohl, D. R., Airasian, P. W., Cruikshank, K. A., Mayer, R. E., Pintrich, P. R., … Wittrock, M. C. (2001). A taxonomy for learning, teaching, and assessing: A revision of Bloom’s taxonomy of educational objectives, abridged edition . White Plains: Longman.
Ates, S., & Cataloglu, E. (2007). The effects of students’ reasoning abilities on conceptual understandings and problem-solving abilities in introductory mechanics. European Journal of Physics , 28 , 1161–1171.
Bagno, E., Eylon, B.-S., & Ganiel, U. (2000). From fragmented knowledge to a knowledge structure: Linking the domains of mechanics and electromagnetism. American Journal of Physics , 68 (S1), S16–S26.
Bailin, S. (1996). Critical thinking. In J. J. Chambliss (Ed.), Philosophy of education: An encyclopedia , (vol. 1671, pp. 119–123). Routledge.
Bangert-Drowns, R. L., & Bankert, E. (1990). Meta-analysis of effects of explicit instruction for critical thinking. Research report. ERIC Number: ED328614.
Google Scholar
Bao, L., Cai, T., Koenig, K., Fang, K., Han, J., Wang, J., … Wu, N. (2009). Learning and scientific reasoning. Science , 323 , 586–587. https://doi.org/10.1126/science.1167740 .
Bao, L., & Redish, E. F. (2001). Concentration analysis: A quantitative assessment of student states. American Journal of Physics , 69 (S1), S45–S53.
Bao, L., & Redish, E. F. (2006). Model analysis: Representing and assessing the dynamics of student learning. Physical Review Special Topics-Physics Education Research , 2 (1), 010103.
Bao, L., Xiao, Y., Koenig, K., & Han, J. (2018). Validity evaluation of the Lawson classroom test of scientific reasoning. Physical Review Physics Education Research , 14 (2), 020106.
Beichner, R. J., Saul, J. M., Abbott, D. S., Morse, J. J., Deardorff, D., Allain, R. J., … Risley, J. S. (2007). The student-centered activities for large enrollment undergraduate programs (SCALE-UP) project. Research-Based Reform of University Physics , 1 (1), 2–39.
Binkley, M., Erstad, O., Herman, J., Raizen, S., Ripley, M., & Rumble, M. (2010). Draft White paper defining 21st century skills . Melbourne: ACTS.
Bloom, B. S., Engelhart, M. D., Furst, E. J., Hill, W. H., & Krathwohl, D. R. (1956). Taxonomy of educational objectives: Handbook 1: Cognitive domain . New York: Longman.
Bransford, J. D., Brown, A. L., & Cocking, R. R. (2000). How people learn , (vol. 11). Washington, DC: National Academy Press.
Brown, A. (1989). Analogical learning and transfer: What develops? In S. Vosniadu, & A. Ortony (Eds.), Similarity and analogical reasoning , (pp. 369–412). New York: Cambridge U.P.
Chapter Google Scholar
Cavallo, A. M. L., Rozman, M., Blickenstaff, J., & Walker, N. (2003). Learning, reasoning, motivation, and epistemological beliefs: Differing approaches in college science courses. Journal of College Science Teaching , 33 (3), 18–22.
Chen, Z., & Klahr, D. (1999). All other things being equal: Acquisition and transfer of the control of variables strategy. Child Development , 70 , 1098–1120.
Chi, M. T., Bassok, M., Lewis, M. W., Reimann, P., & Glaser, R. (1989). Self-explanations: How students study and use examples in learning to solve problems. Cognitive Science , 13 (2), 145–182.
Chi, M. T., Feltovich, P. J., & Glaser, R. (1981). Categorization and representation of physics problems by experts and novices. Cognitive Science , 5 (2), 121–152.
Chi, M. T., & Slotta, J. D. (1993). The ontological coherence of intuitive physics. Cognition and Instruction , 10 (2–3), 249–260.
Chi, M. T., Slotta, J. D., & De Leeuw, N. (1994). From things to processes: A theory of conceptual change for learning science concepts. Learning and Instruction , 4 (1), 27–43.
Chi, M. T. H. (1992). Conceptual change within and across ontological categories: Examples from learning and discovery in science. In R. N. Giere (Ed.), Cognitive models of science . Minneapolis: University of Minnesota Press.
Chiu, M. H. (2001). Algorithmic problem solving and conceptual understanding of chemistry by students at a local high school in Taiwan. Proceedings-National Science Council Republic of China Part D Mathematics Science and Technology Education , 11 (1), 20–38.
Chiu, M.-H., Guo, C. J., & Treagust, D. F. (2007). Assessing students’ conceptual understanding in science: An introduction about a national project in Taiwan. International Journal of Science Education , 29 (4), 379–390.
Clement, J. (1982). Students’ preconceptions in introductory mechanics. American Journal of Physics , 50 (1), 66–71.
Coletta, V. P., & Phillips, J. A. (2005). Interpreting FCI scores: Normalized gain, preinstruction scores, and scientific reasoning ability. American Journal of Physics , 73 (12), 1172–1182.
Cracolice, M. S., Deming, J. C., & Ehlert, B. (2008). Concept learning versus problem solving: A cognitive difference. Journal of Chemical Education , 85 (6), 873.
De Jong, T., & Ferguson-Hesler, M. G. M. (1986). Cognitive structure of good and poor problem solvers in physics. Journal of Educational Psychology , 78 , 279–288.
DiSessa, A. A. (1993). Toward an epistemology of physics. Cognition and Instruction , 10 (2–3), 105–225.
Duit, R., & Treagust, D. F. (2003). Conceptual change: A powerful framework for improving science teaching and learning. International Journal of Science Education , 25 (6), 671–688.
Dykstra Jr., D. I., Boyle, C. F., & Monarch, I. A. (1992). Studying conceptual change in learning physics. Science Education , 76 (6), 615–652.
Ennis, R. (1993). Critical thinking assessment. Theory Into Practice , 32 (3), 179–186.
Etkina, E., & Van Heuvelen, A. (2001). Investigative science learning environment: Using the processes of science and cognitive strategies to learn physics. In Proceedings of the 2001 physics education research conference , (pp. 17–21). Rochester.
Etkina, E., Van Heuvelen, A., White-Brahmia, S., Brookes, D. T., Gentile, M., Murthy, S., … Warren, A. (2006). Scientific abilities and their assessment. Physical Review Special Topics-Physics Education Research , 2 (2), 020103.
Eylon, B.-S., & Reif, F. (1984). Effects of knowledge organization on task performance. Cognition and Instruction , 1 (1), 5–44.
Fabby, C., & Koenig, K. (2013). Relationship of scientific reasoning to solving different physics problem types. In Proceedings of the 2013 Physics Education Research Conference, Portland, OR .
Facione, P. A. (1990). Critical thinking: A statement of expert consensus for purposes of educational assessment and instruction – The Delphi report . Millbrae: California Academic Press.
Ferguson-Hesler, M. G. M., & De Jong, T. (1990). Studying physics texts: Differences in study processes between good and poor solvers. Cognition and Instruction , 7 (1), 41–54.
Fisher, A. (2001). Critical thinking: An introduction . Cambridge: Cambridge University Press.
Freeman, S., Eddy, S. L., McDonough, M., Smith, M. K., Okoroafor, N., Jordt, H., & Wenderoth, M. P. (2014). Active learning increases student performance in science, engineering, and mathematics. Proceedings of the National Academy of Sciences , 111 (23), 8410–8415.
Glaser, E. M. (1941). An experiment in the development of critical thinking . New York: Teachers College, Columbia University.
Hake, R. R. (1992). Socratic pedagogy in the introductory physics laboratory. The Physics Teacher , 30 , 546.
Hake, R. R. (1998). Interactive-engagement versus traditional methods: A six-thousand-student survey of mechanics test data for introductory physics courses. American Journal of Physics , 66 (1), 64–74.
Halloun, I. A., & Hestenes, D. (1985a). The initial knowledge state of college physics students. American Journal of Physics , 53 (11), 1043–1055.
Halloun, I. A., & Hestenes, D. (1985b). Common sense concepts about motion. American Journal of Physics , 53 (11), 1056–1065.
Halpern, D. F. (1999). Teaching for critical thinking: Helping college students develop the skills and dispositions of a critical thinker. New Directions for Teaching and Learning , 80 , 69–74. https://doi.org/10.1002/tl.8005 .
Hardiman, P. T., Dufresne, R., & Mestre, J. P. (1989). The relation between problem categorization and problem solving among experts and novices. Memory & Cognition , 17 (5), 627–638.
Heller, J. I., & Reif, F. (1984). Prescribing effective human problem-solving processes: Problem description in physics. Cognition and Instruction , 1 (2), 177–216.
Hoellwarth, C., Moelter, M. J., & Knight, R. D. (2005). A direct comparison of conceptual learning and problem solving ability in traditional and studio style classrooms. American Journal of Physics , 73 (5), 459–462.
Hofstein, A., & Lunetta, V. N. (1982). The role of the laboratory in science teaching: Neglected aspects of research. Review of Educational Research , 52 (2), 201–217.
Hsu, L., Brewe, E., Foster, T. M., & Harper, K. A. (2004). Resource letter RPS-1: Research in problem solving. American Journal of Physics , 72 (9), 1147–1156.
Jensen, J. L., & Lawson, A. (2011). Effects of collaborative group composition and inquiry instruction on reasoning gains and achievement in undergraduate biology. CBE - Life Sciences Education , 10 , 64–73.
Johnson, M. A., & Lawson, A. E. (1998). What are the relative effects of reasoning ability and prior knowledge on biology achievement in expository and inquiry classes? Journal of Research in Science Teaching , 35 (1), 89–103.
Kalman, C., & Lattery, M. (2018). Three active learning strategies to address mixed student epistemologies and promote conceptual change. Frontiers in ICT , 5 (19), 1–9.
Karplus, R. (1964). The science curriculum improvement study. Journal of College Science Teaching , 2 (4), 293–303.
Kim, E., & Pak, S.-J. (2002). Students do not overcome conceptual difficulties after solving 1000 traditional problems. American Journal of Physics , 70 (7), 759–765.
Koenig, K., Schen, M., & Bao, L. (2012). Explicitly targeting pre-service teacher scientific reasoning abilities and understanding of nature of science through an introductory science course. Science Educator , 21 (2), 1–9.
Koenig, K., Schen, M., Edwards, M., & Bao, L. (2012). Addressing STEM retention through a scientific thought and methods course. Journal of College Science Teaching , 41 , 23–29.
Koenig, K., Wood, K., Bortner, L., & Bao, L. (2019). Modifying traditional labs to target scientific reasoning. Journal of College Science Teaching , 48 (5), 28-35.
Kozminski, J., Beverly, N., Deardorff, D., Dietz, R., Eblen-Zayas, M., Hobbs, R., … Zwickl, B. (2014). AAPT recommendations for the undergraduate physics laboratory curriculum , (pp. 1–29). American Association of Physics Teachers Retrieved from https://www.aapt.org/Resources/upload/LabGuidlinesDocument_EBendorsed_nov10.pdf .
Kuhn, D. (1992). Thinking as argument. Harvard Educational Review , 62 (2), 155–178.
Larkin, J., McDermott, J., Simon, D. P., & Simon, H. A. (1980). Expert and novice performance in solving physics problems. Science , 208 (4450), 1335–1342.
Laws, P. W. (2004). Workshop physics activity guide, module 4: Electricity and magnetism. In Workshop physics activity guide . Wiley-VCH.
Lawson, A. E. (1978), The development and validation of a classroom test of formal reasoning, Journal of Research in Science Teaching , 15 (1), 11–24.
Lawson, A. E. (1992). The development of reasoning among college biology students - a review of research. Journal of College Science Teaching , 21 , 338–344.
Lawson, A. E. (2000). Classroom test of scientific reasoning: Multiple choice version, based on Lawson, A. E. 1978. Development and validation of the classroom test of formal reasoning. Journal of Research in Science Teaching , 15 (1), 11–24.
Lee, H. S., Liu, O. L., & Linn, M. C. (2011). Validating measurement of knowledge integration in science using multiple-choice and explanation items. Applied Measurement in Education , 24 (2), 115–136.
Linn, M. C. (2005). The knowledge integration perspective on learning and instruction. In R. K. Sawyer (Ed.), The Cambridge handbook of the learning sciences , (pp. 243–264). Cambridge: Cambridge University Press. https://doi.org/10.1017/CBO9780511816833.016 .
Lipman, M. (2003). Thinking in education , (2nd ed., ). Cambridge: Cambridge University Press.
Liu, X. (2010). Science and engineering education sources. Using and developing measurement instruments in science education: A Rasch modeling approach . Charlotte: IAP Information Age Publishing.
Marzano, R. J., Brandt, R. S., Hughes, C. S., Jones, B. F., Presseisen, B. Z., Rankin, S. C., et al. (1988). Dimensions of thinking, a framework for curriculum and instruction . Alexandria: Association for Supervision and Curriculum Development.
Mazur, E. (1997). Peer instruction: A user’s manual . Upper Saddle River: Prentice Hall.
McDermott, L. C. (1996). Physics by Inquiry: An Introduction to the Physical Sciences . John Wiley & Sons, New York, NY.
McDermott, L. C., & Redish, E. F. (1999). Resource letter: PER-1: Physics education research. American Journal of Physics , 67 (9), 755–767.
McKeachie, W. J. (1986). Teaching and learning in the college classroom: A review of the research literature . Ann Arbor: National Center for Research to Improve Postsecondary Teaching and Learning.
Meltzer, D. E., & Otero, V. K. (2015). A brief history of physics education in the United States. American Journal of Physics , 83 (5), 447–458.
Meltzer, D. E., & Thornton, R. K. (2012). Resource letter ALIP-1: Active-learning instruction in physics. American Journal of Physics , 80 (6), 478–496.
Minstrell, J. (1992). Facets of students’ knowledge and relevant instruction. In R. Duit, F. Goldberg, & H. Niedderer (Eds.), Proceedings of the international workshop: Research in physics learning- theoretical issues and empirical studies , (pp. 110–128). The Institute for Science Education.
Nakhleh, M. B. (1993). Are our students conceptual thinkers or algorithmic problem solvers? Identifying conceptual students in general chemistry. Journal of Chemical Education , 70 (1), 52. https://doi.org/10.1021/ed070p52 .
Nakhleh, M. B., & Mitchell, R. C. (1993). Concept learning versus problem solving: There is a difference. Journal of Chemical Education , 70 (3), 190. https://doi.org/10.1021/ed070p190 .
National Research Council (2011). Assessing 21st century skills: Summary of a workshop . Washington, DC: The National Academies Press. https://doi.org/10.17226/13215 .
Book Google Scholar
National Research Council (2012a). Education for life and work: Developing transferable knowledge and skills in the 21st century . Washington, DC: The National Academies Press.
National Research Council (2012b). A framework for K-12 science education: Practices, crosscutting concepts, and core ideas . Washington, DC: National Academies Press.
National Research Council (2012c). Discipline-based education research: Understanding and improving learning in undergraduate science and engineering . Washington, DC: National Academies Press.
National Science & Technology Council (2018). Charting a course for success: America’s strategy for STEM education . Washington, DC: Office of Science and Technology Policy.
NCET. (1987). Critical thinking as defined by the National Council for excellence in critical thinking, statement by Michael Scriven & Richard Paul, presented at the 8th annual conference on critical thinking and education reform. Retrieved December 4, 2018, from http://www.criticalthinking.org/pages/defining-critical-thinking/766 .
Nordine, J., Krajcik, J., & Fortus, D. (2011). Transforming energy instruction in middle school to support integrated understanding and future learning. Science Education , 95 (4), 670–699.
Nurrenbern, S. C., & Pickering, M. (1987). Concept learning versus problem solving: Is there a difference? Journal of Chemical Education , 64 (6), 508.
Paul, R. (1990). Critical thinking: What every person needs to survive in a rapidly changing world . Rohnert Park: Center for Critical Thinking and Moral Critique.
Perkins, D. N., & Salomon, G. (1989). Are cognitive skills context-bound? Educational Researcher , 18 (1), 16–25.
Posner, G., Strike, K., Hewson, P., & Gertzog, W. (1982). Accommodation of a scientific conception: Toward a theory of conceptual change. Science Education , 66 (2), 211–227.
Reay, N. W., Bao, L., Li, P., Warnakulasooriya, R., & Baugh, G. (2005). Toward the effective use of voting machines in physics lectures. American Journal of Physics , 73 (6), 554–558.
Reimers, F. M., & Chung, C. K. (Eds.) (2016). Teaching and learning for the twenty-first century: Educational goals, policies and curricula from six nations . Cambridge: Harvard Education Press.
Salomon, G., & Perkins, D. N. (1989). Rocky roads to transfer: Rethinking mechanism of a neglected phenomenon. Educational Psychologist , 24 (2), 113–142.
Schoenfeld, A. H., & Herrmann, D. J. (1982). Problem perception and knowledge structure in expert and novice mathematical problem solvers. Journal of Experimental Psychology: Learning, Memory, and Cognition , 8 (5), 484.
Shaw, V. F. (1996). The cognitive processes in informal reasoning. Thinking and Reasoning , 2 (1), 51–80.
She, H., & Liao, Y. (2010). Bridging scientific reasoning and conceptual change through adaptive web-based learning. Journal of Research in Science Teaching , 47 (1), 91–119.
Shen, J., Liu, O. L., & Chang, H.-Y. (2017). Assessing students’ deep conceptual understanding in physical sciences: An example on sinking and floating. International Journal of Science and Mathematics Education , 15 (1), 57–70. https://doi.org/10.1007/s10763-015-9680-z .
Siegel, H. (1988). Educating reason: Rationality, critical thinking and education , (vol. 1). New York: Routledge.
Slotta, J. D., Chi, M. T., & Joram, E. (1995). Assessing students’ misclassifications of physics concepts: An ontological basis for conceptual change. Cognition and Instruction , 13 (3), 373–400.
Smith III, J. P., DiSessa, A. A., & Roschelle, J. (1994). Misconceptions reconceived: A constructivist analysis of knowledge in transition. The Journal of the Learning Sciences , 3 (2), 115–163.
Smith, M. U. (1992). Expertise and organization of knowledge: Unexpected differences among genetic counselors, faculty members and students on problem categorization tasks. Journal of Research in Science Teaching , 29 (2), 179–205.
Sobhanzadeh, M., Kalman, C. S., & Thompson, R. I. (2017). Labatorials in introductory physics courses. European Journal of Physics , 38 , 1–18.
Sokoloff, D. R., Thornton, R. K., & Laws, P. W. (2011). RealTime physics: Active learning laboratories . New York: Wiley.
Stamovlasis, D., Tsaparlis, G., Kamilatos, C., Papaoikonomou, D., & Zarotiadou, E. (2005). Conceptual understanding versus algorithmic problem solving: Further evidence from a national chemistry examination. Chemistry Education Research and Practice , 6 (2), 104–118.
Tanenbaum, C. (2016). STEM 2026: A vision for innovation in STEM education . Washington, DC: US Department of Education.
Thornton, R. K., & Sokoloff, D. R. (1998). Assessing student learning of Newton’s laws: The force and motion conceptual evaluation and the evaluation of active learning laboratory and lecture curricula. American Journal of Physics , 66 (4), 338–352.
Trowbridge, L. W., Bybee, R. W., & Powell, J. C. (2000). Teaching secondary school science: Strategies for developing scientific literacy . Upper Saddle River: Merrill-Prentice Hall.
United States Chamber of Commerce (2017). Bridging the soft skills gap: How the business and education sectors are partnering to prepare students for the 21 st century workforce . Washington DC: Center for Education and Workforce, U.S. Chamber of Commerce Foundation.
Veldhuis, G. H. (1990). The use of cluster analysis in categorization of physics problems. Science Education , 74 (1), 105–118.
Vosniadou, S., Vamvakoussi, X., & Skopeliti, I. (2008). The framework theory approach to the problem of conceptual change. In S. Vosniadou (Ed.), International handbook of research on conceptual change . New York: Routledge.
Wexler, P. (1982). Structure, text, and subject: A critical sociology of school knowledge. In M. W. Apple (Ed.), Cultural and economic reproduction in education: Essays on class, ideology and the state . London: Routledge & Regan Paul.
Zeineddin, A., & Abd-El-Khalick, F. (2010). Scientific reasoning and epistemological commitments: Coordination of theory and evidence among college science students. Journal of Research in Science Teaching , 47 (9), 1064–1093.
Zimmerman, C. (2000). The development of scientific reasoning skills. Developmental Review , 20 (1), 99–149.
Download references
Acknowledgements
The research is supported in part by NSF Awards DUE-1431908 and DUE-1712238. Any opinions, findings, and conclusions or recommendations expressed in this paper are those of the author(s) and do not necessarily reflect the views of the National Science Foundation.
The research is supported in part by NSF Awards DUE-1431908 and DUE-1712238.
Author information
Authors and affiliations.
The Ohio State University, Columbus, OH, 43210, USA
University of Cincinnati, Cincinnati, OH, 45221, USA
Kathleen Koenig
You can also search for this author in PubMed Google Scholar
Contributions
LB developed the concept, wrote a significant portion of the review and position, and synthesized the paper. KK wrote and edited a significant portion of the paper. Both authors read and approved the final manuscript.
Corresponding author
Correspondence to Lei Bao .
Ethics declarations
Competing interests.
The authors declare that they have no competing interests.
Additional information
Publisher’s note.
Springer Nature remains neutral with regard to jurisdictional claims in published maps and institutional affiliations.
Rights and permissions
Open Access This article is distributed under the terms of the Creative Commons Attribution 4.0 International License ( http://creativecommons.org/licenses/by/4.0/ ), which permits unrestricted use, distribution, and reproduction in any medium, provided you give appropriate credit to the original author(s) and the source, provide a link to the Creative Commons license, and indicate if changes were made.
Reprints and permissions
About this article
Cite this article.
Bao, L., Koenig, K. Physics education research for 21 st century learning. Discip Interdscip Sci Educ Res 1 , 2 (2019). https://doi.org/10.1186/s43031-019-0007-8
Download citation
Received : 17 April 2019
Accepted : 13 June 2019
Published : 28 November 2019
DOI : https://doi.org/10.1186/s43031-019-0007-8
Share this article
Anyone you share the following link with will be able to read this content:
Sorry, a shareable link is not currently available for this article.
Provided by the Springer Nature SharedIt content-sharing initiative
- Twenty-first century learning
- STEM education
- Scientific reasoning
- Deep learning

Physics & Astronomy
- 1: Explore Topic
- 2: Find Sources
- 3: Evaluate
- Last Updated: Mar 22, 2024 1:33 PM
- URL: https://libguides.unco.edu/physics

PHYS 354: Medical Physics Principles: Sources + Literature Review
- Biomedical Optics
- Biomedical Technology
- General Medicine + Biology
- Magnetic Resonance Imaging
- Nuclear Medicine
- Radiation Therapy
- Radiology + Medical Physics
- Ultrasound & Photoacoustics
- Medical Physics Resources
- Physics Citations
- Summarizing Research Articles & Annotated Bibliographies
- Sources + Literature Review
- Reference Management
- KU Library Resources
What is a Literature Review?
A literature review is a comprehensive study and interpretation of literature that addresses a specific topic.

Literature reviews are generally conducted in one of two ways:
1) As a preliminary review before a larger study in order to critically evaluate the current literature and justify why further study and research is required.
2) As a project in itself that provides a comprehensive survey of the works published in a particular discipline or area of research over a specified period of time.
Why conduct a literature review? They provide you with a handy guide to a particular topic. If you have limited time to conduct research, literature reviews can give you an overview or act as a stepping stone.
More: different types of literature reviews on how to conduct a literature review.
Literature Review Links
- PhD on Track: Types of Reviews Narrative & Systematic
- Purdue Owl: Literature Reviews
- Purdue OWL: Writing a Literature Review
How to Develop a Literature Review
How to develop a literature review from Academic Research Foundations: Quantitative by Rolin Moe
What is the Difference Between a Systematic Review and a Meta-analysis?
Dr. Singh discusses the difference between a systematic review and a meta-analysis.
Library Resources
Purpose of a Literature Review
Purpose of a literature review from Academic Research Foundations: Quantitative by Rolin Moe
- << Previous: Summarizing Research Articles & Annotated Bibliographies
- Next: Presenting >>
- Last Updated: Mar 7, 2024 1:24 PM
- URL: https://libguides.kettering.edu/PHYS354
Physical Review E
Covering statistical, nonlinear, biological, and soft matter physics.
- Collections
- Editorial Team
Unifying model for the rheological behavior of hygroresponsive materials
Júlio o. amando de barros and falk k. wittel, phys. rev. e 109 , 044139 – published 17 april 2024.
- No Citing Articles
- INTRODUCTION
- MATERIALS AND METHODS
- RESULTS AND DISCUSSION
- CONCLUSIONS
- ACKNOWLEDGMENTS
Hygroresponsive materials exhibit a complex structure-to-property relationship. The interactions of water within these materials under varying hygric and mechanical loads play a crucial role in their macroscopic deformation and final application. While multiple models are available in literature, many lack a comprehensive physical understanding of these phenomena. In this paper, we introduce a stick-slip fiber bundle model that captures the fundamental behaviors of hygroresponsive materials. We incorporate moisture-dependent elements and rules governing the initiation and relaxation of slip strains as well as failure to the statistical approach offered by fiber bundle models. The additional features are based on well-founded interpretations of the structure-to-property relationship in cellulosic materials. Slip strains are triggered by changes in load and moisture, as well as by creep deformations. When subjected to moisture cycles, the model accumulates slip strains, resulting in mechanosorptive behavior. When the load is removed, slip strains are partially relaxed, and subsequent moisture cycles trigger further relaxation, as expected from observations with mechanosorptive material. Importantly, these slip strains are not considered plastic strains; instead, they are unified, nonlinear frozen strains, activated by various stimuli. Failure of fibers is defined by a critical number of slip events allowing for an integrated simulation from intact, via damaged, failed states. We investigate the transition between these regimes upon changes in the hygric and mechanical loading history for relevant parameter ranges. Our enhanced stick-slip fiber bundle model increases the understanding of the intricate behavior of hygroresponsive materials and contributes to a more robust framework for analyzing and interpreting their properties.
- Received 8 November 2023
- Accepted 13 March 2024
DOI: https://doi.org/10.1103/PhysRevE.109.044139
©2024 American Physical Society
Physics Subject Headings (PhySH)
- Research Areas
Authors & Affiliations
- Institute for Building Materials, ETH Zurich, Leopold-Ruzicka-Weg 4, 8093 Zurich, Switzerland
- * [email protected]
- † [email protected]
Article Text (Subscription Required)
References (subscription required).
Vol. 109, Iss. 4 — April 2024
Access Options
- Buy Article »
- Log in with individual APS Journal Account »
- Log in with a username/password provided by your institution »
- Get access through a U.S. public or high school library »
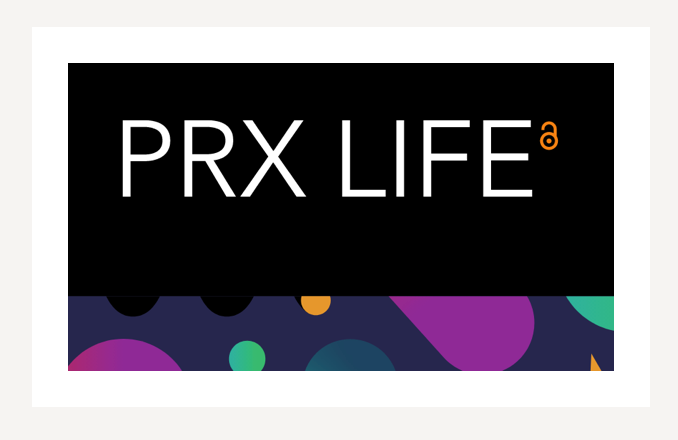
Authorization Required
Other options.
- Buy Article »
- Find an Institution with the Article »
Download & Share
Main features observed in hygroresponsive materials. The strain ( ɛ ) is presented in black, while the load ( σ ) and relative humidity (RH) are represented by red, respectively, blue dashed lines. Letters indicate different features like H for hygroexpansion, E for elasticity, VE for viscoelasticity, and MS for mechanosorption.
Micromechanical interpretation of stick-slip dynamics on wood fibers. We present a wood fiber on the left with its microfibril aggregates exposed. Following to the right, the aggregates' interactions and their behavior under mechanical and moisture stimuli are presented. ɛ d and ɛ w are the nonslip strain at the dry state and wet state, respectively, while ɛ d S , ɛ w S , and ɛ t S are the slip strains respective to drying, wetting, and time evolution.
Sketch of the FBM with green representing slip, yellow tensile, and red compressive strains. On the right is the rheological representation of a single fiber model with elastic, viscoelastic, hygro-expansive, and slip element in series. The total strain ɛ is present in both representations.
Flowchart of implemented code.
SS-FBM normalized strain over the normalized time. Solid lines represent the normalized strain ɛ / ɛ c , while dashed lines represent the normalized strain without slip ( ɛ − 〈 ɛ S 〉 ) / ɛ c . Line colors resemble softening ratios D w / D d .
Residual normalized stresses (a) and number of slip events (b) for each fiber of the bundle at t = 40 τ in Fig. 5 .
SS-FBM strain over five moisture cycles. Solid lines represent total values of ɛ and ɛ S in both plots, respectively, while dashed lines represent their labeled components.
SS-FBM slip strain evolution over ten moisture cycles and the respective normalized slip (green) and reverse slip (black) avalanches.
Probability density of slip thresholds for increasing moisture cycles MC with identical legend.
SS-FBM maximum strain-stress relation for different dry-to-wet compliance ratios and comparison to the strength with the dry FBM (inset).
Applied load over the number of cycles that can be imposed before the failure of the system. If the system doesn't fail, the maximum number of imposed cycles is 50.
Comparison of the proposed model (SS-FBM) results to empirical data and the hygrolock modeling approach proposed by Dubois et al. [ 42 ].
Sign up to receive regular email alerts from Physical Review E
- Forgot your username/password?
- Create an account
Article Lookup
Paste a citation or doi, enter a citation.
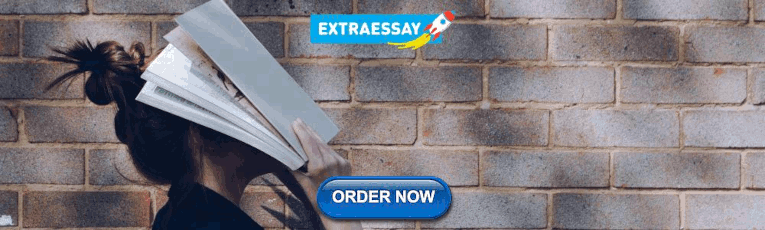
IMAGES
VIDEO
COMMENTS
A literature review surveys scholarly articles, books and other sources (e.g. dissertations, conference proceedings) relevant to a particular issue, area of research, or theory, providing a description, summary, and critical evaluation of each work.. Purpose. Provide context for a research paper; Explore the history and development of a topic; Examine the scholarly conversation surrounding the ...
This video will provide a short introduction to literature reviews. Steps For Writing a Literature Review. Recommended steps for writing a literature review: Review what a literature review is, and is not. Review your assignment and seek clarification from your instructor if needed. Narrow your topic. Search and gather literature resources.
Notion is an organization application that allows you to make various pages and databases. It's kind of like your own personal wiki- you can link your pages and embed databases into another page, adding filters and sorting them using user-set properties. The databases are what I use the most.
The best proposals are timely and clearly explain why readers should pay attention to the proposed topic. It is not enough for a review to be a summary of the latest growth in the literature: the ...
This Physical Review Physics Education Research (PRPER) Focused Collection was curated to bring to light curriculum design decisions and the factors that shape them. By making decisions about design explicit, we can better understand the contexts behind our research claims, hold curricula up to informed critique, and support new scholars as ...
Choosing a Topic. Choosing your topic is one of the most important steps for a graduate student, and should be done in consultation with your faculty advisor. Some of the tips presented in the video below can help you get started.
A scoping review employs the systematic review methodology to explore a broader topic or question rather than a specific and answerable one, as is generally the case with a systematic review. Authors of these types of reviews seek to collect and categorize the existing literature so as to identify any gaps. Rapid Review
A literature review is a comprehensive study and interpretation of literature that addresses a specific topic.. Literature reviews are generally conducted in one of two ways: 1) As a preliminary review before a larger study in order to critically evaluate the current literature and justify why further study and research is required.
At Nature Reviews, editors work closely with authors and referees to create high-quality, timely and accessible resources for the scientific community. Nature Reviews Physics is an online-only ...
Reviews of Modern Physics (RMP) brings the broad fundamental physics literature in established topical areas together and places it within the context of current trends in research and applications. Its in-depth review articles and shorter Colloquia serve students, engineers, and physics researchers in a range of fields.
Eye tracking is becoming increasingly popular in physics education research (PER). As technology has advanced considerably in recent years and has become more user friendly, it is anticipated that eye tracking will play an increasingly significant role in assessing student learning at the process level in future studies.
Effect of integrating physics education technology simulations on students' conceptual understanding in physics: A review of literature Herbert James Banda and Joseph Nzabahimana Phys. Rev. Phys. Educ. Res. 17, 023108 - Published 21 December 2021
A literature review is a comprehensive study and interpretation of literature that addresses a specific topic.. Literature reviews are generally conducted in one of two ways: 1) As a preliminary review before a larger study in order to critically evaluate the current literature and justify why further study and research is required.
Abstract. The Review summarizes much of particle physics and cosmology. Using data from previous editions, plus 2,143 new measurements from 709 papers, we list, evaluate, and average measured properties of gauge bosons and the recently discovered Higgs boson, leptons, quarks, mesons, and baryons. We summarize searches for hypothetical particles ...
Phys. Rev. Phys. Educ. Res. 18, 010136 (2022) - Published 2 May 2022. Retrieval practice of physics principles and their conditions of application before self-explanation can have a positive effect on posttest problem-solving scores and can increase the quality of students' self-explanations.
Reviews in Physics (REVIP) is a gold open access journal publishing high-quality papers in all areas of applied and fundamental physics.REVIP aims to provide a platform for researchers who wish to summarize a field, report on their own progress, provide a forward-looking perspective of the future directions and challenges or discuss important ideas and controversies of current interest.
This paper performs a systematic literature review on physics-based modeling techniques, focusing on assessing energy conservation measures. Different UBEM case studies are examined based on the number and type of buildings, building systems, occupancy schedule modeling, archetype development, weather data type, and model calibration methods. ...
Systematic Literature Review: Quantum Machine Learning and its applications. David Peral García, Juan Cruz-Benito, Francisco José García-Peñalvo. Quantum computing is the process of performing calculations using quantum mechanics. This field studies the quantum behavior of certain subatomic particles for subsequent use in performing ...
review, I have primarily concentrated on research conducted on secondary and college teachers; however, I have also included some studies conducted on teachers of primary grades when they are particularly relevant. The second part of this literature review is a briefsummary of research on the effective teaching of physics problem solving.
Physics: Literature Review. Welcome to the guide for the Physics. Here you'll find resources and information that will assist you with your research. Click on the tabs on this page to uncover detailed lists of information sources to help answer your questions. Home.
This paper provides a brief review of physics education research as a means for providing a context towards future work in promoting deep learning and fostering abilities in high-end reasoning. Through a synthesis of the literature around twenty-first Century skills and physics education, a set of concretely defined education and research goals ...
Physics & Astronomy. This page is not currently available due to visibility settings. Last Updated: Mar 22, 2024 1:33 PM. URL: https://libguides.unco.edu/physics.
A literature review is a comprehensive study and interpretation of literature that addresses a specific topic.. Literature reviews are generally conducted in one of two ways: 1) As a preliminary review before a larger study in order to critically evaluate the current literature and justify why further study and research is required.
Hygroresponsive materials exhibit a complex structure-to-property relationship. The interactions of water within these materials under varying hygric and mechanical loads play a crucial role in their macroscopic deformation and final application. While multiple models are available in literature, many lack a comprehensive physical understanding of these phenomena. In this paper, we introduce a ...