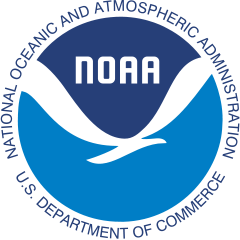
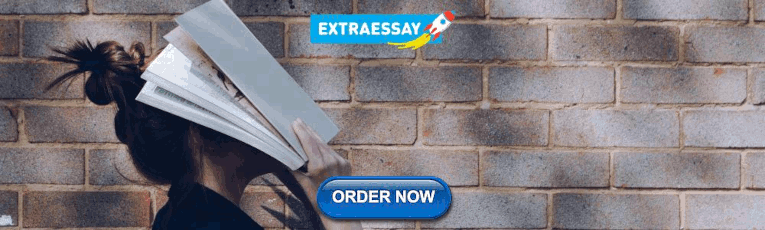
Sahel Drought: Understanding the Past and Projecting into the Future
The Sahel occupies a transitional zone between the Sahara to the north and tropical rain forests to the south. As for many such transitional zones adjacent to tropical rain belts, climate is highly variable due to the sharp gradients in rainfall. In the 1970’s and 1980’s, the region experienced a profound drought, with over a 30% decrease in rainfall over most of the Sahel as compared to the 1950’s– arguably the most dramatic drought in any region of this large an extent observed in the 20th century. In the figure below, the black line is the observed summer (July-Aug-September) rainfall averaged over the Sahel, normalized by its mean over the time interval shown, taken from the University of East Anglia’s Climate Research Unit ( CRUTS2.1 ). A defense of the quality of the observational record after 1930 is provided by Dai, et. al.
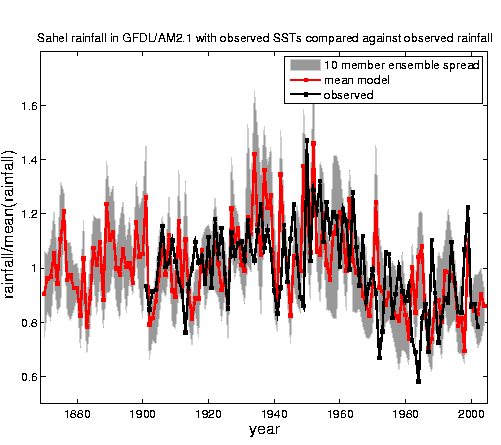
Given this potential for large rainfall variations, and the economic, political, and social challenges facing the region, the possibility that increasing greenhouse gases could alter Sahelian rainfall is of great concern. Understanding the Sahel drought of the late 20th century and improving our future projections for the hydrological cycle in this region is a major challenge for climate dynamics and climate modeling.
It has been understood since the 1980’s ( Folland, Palmer, and Parker ) that changes in ocean temperature played a significant role in producing the late 20th century Sahel drought. For a more recent influential paper making a strong case for the dominance of oceanic forcing, see Giannini, Saravanan, and Chang . GFDL’s AM2.1/LM2 atmosphere/land model, developed in part for the IPCC’s 4th Assessment, produces the result shown above when provided with the observed ocean surface temperatures over the 20th century. This computation was repeated 10 times — the gray area is the full spread of these 10 simulations, while the red line is the mean. The impressive agreement extends to the beginning of the century, which is somewhat surprising given the uncertainties in the early decades of the 20th century in both the rainfall record and the ocean temperatures used as a boundary condition for these simulations. This result is described in Held, Delwortih, Lu, Findell, and Knutson. The observation that the rainfall reaches a maximum in mid-century, and that this maximum can be simulated, given the observed ocean surface temperatures, is evidence that the drying from the 1950’s to the 1980’s is unlikely to be primarily forced by increasing greenhouse gases in the atmosphere (assuming that these early century rainfall and ocean temperature records are robust).
A variety of modeling studies have pointed to changes in the inter-hemispheric temperature gradient, within the tropical oceans but also globally, as being of key importance for Sahel rainfall, with drought occurring when the Northern Hemisphere oceans are relatively cold as compared to the Southern Hemisphere oceans. During the early part of the 20th century, the Northern Hemisphere warmed more rapidly than the Southern Hemisphere, but from mid-century till 1980, the Northern Hemisphere cooled on average, while the Southern Hemisphere continued to warm. Since 1980, the Northern Hemisphere has once again warmed more rapidly. The result, as illustrated below by the temperature record (land plus ocean) as compiled by NASA’s Goddard Institute for Space Studies, the maximum warmth of the Southern relative to the Northern Hemisphere was achieved during the most severe period of the Sahel drought.
The picture that emerges seems to be relatively simple, even though the underlying dynamics may be quite complex: the tropical rain-belts are attracted to the relatively warm hemisphere, with the Sahel, which we can think of as the northernmost extension of these rain-belts in Northern summer, suffering drought when the Southern Hemisphere is relatively warm. This effect is thought to be relevant more generally throughout the tropics, and not just for Africa. See, for example, Chiang and Bitz on the interaction between the Ice Age ice sheets and tropical precipitation, and Yoshimori and Broccoli on the consequences for the tropical rain-belts of north-south asymmetry in radiative forcing. Sarah Kang (graduate student in Princeton’s AOS Program), has studied the underlying dynamics in a variety of idealized models (the first paper emerging from this work is Kang, Held, Frierson and Zhao ).
An alternative hypothesis, popular during the 1970-1980’s drought itself, is modification of the land surface due to human activity, usually termed “desertification”. Climate models do suggest that one can reduce Sahel rainfall by increasing the surface albedo (the reflectivity of the surface to solar radiation), a result expected from the loss of vegetation, but models have not produced responses of adequate magnitude by this mechanism, and it is difficult to explain a mid-century maximum in rainfall through land alteration. See Brooks for further discussion of this major shift in perspective from one in which land alteration is directly responsible for the drought to one in which oceanic changes unrelated to any land modifications are deemed to be the dominant cause. The dominance of oceanic forcing in the past does not deny the possibility that major land modifications could be a factor in the future, or that the feedback of land vegetation to the changes in rainfall could be important for the observed variations.
If we accept that inter-hemispheric gradients in ocean temperature are a key proximal cause of multi-decadal Sahel rainfall variations, the search for the ultimate cause becomes the search for an explanation for these ocean temperature variations, A substantial part of these changes in temperature gradient is likely due to internal variability, the source of this variability most likely being in the meridional overturning circulation in the Atlantic Ocean. Zhang and Delworth , for example, have shown how manipulating the northward heat flux by the Atlantic ocean in a climate model can alter Sahel rainfall. But aerosol forcing has also been implicated as a potential candidate for the drying tendency since mid-century, with increasing sulphate pollution in the Northern Hemisphere causing the Northern Hemisphere to cool relative to the south. The recent warming in the north is, in this interpretation, caused by cleansing of the Northern Hemisphere atmosphere. Rotstayn and Lohmann have argued that the evolution of aerosol forcing is large enough to explain a large fraction of the Sahel drought, if one includes relatively large indirect aerosol effects associated with cloud/aerosol interactions. Biasutti and Giannini describe the results in the CMIP3 coupled-climate model simulations utilized by the IPCC’s 4th Assessment, and conclude that there is a small forced component to the drying trend over the 20th century in most of these models. This trend does not continue into the future in the multi-model average, implying that the dominant forcing in the models is not greenhouse gases but rather aerosols, in qualitative agreement with Rotstayn and Lohmann. Greater confidence in our ability to estimate past variations in aerosol forcing will be needed to make further progress on this aspect of the problem. But, once again, to the extent that the precipitation did indeed maximize in mid-century, the more plausible explanation for the bulk of the observed variability remains internal variability.
CM2.1 — an outlier in its prediction of the future of Sahel rainfall
Closer comparison of the changes in the inter-hemispheric ocean temperature gradient and Sahel rainfall suggests the possibility that another process has operated in recent years. The Northern Hemisphere has warmed quite rapidly since 1980, which one might expect to have led to a more pronounced amelioration of the drought. Indeed. Atlantic hurricanes, which are plausibly controlled by the same difference in temperatures (at least within the Atlantic Ocean) have recovered to an activity level more comparable to mid-century values.
Analysis of the AM2.1 atmospheric model, which generates the striking fit to observed Sahel rainfall variations shown above, when forced with observed surface ocean temperatures, shows that the model is not simply responding to changes in inter-hemispheric temperature gradients. The model also dries the Sahel when the oceans are warmed uniformly. Given this behavior, it is not surprising that when this model is coupled to an ocean in GFDL’s CM2 climate model, it predicts continued drying into the future; indeed, as indicated in the following figure, the drying it projects exceeds that experienced in the 1980’s by the mid-21st century.
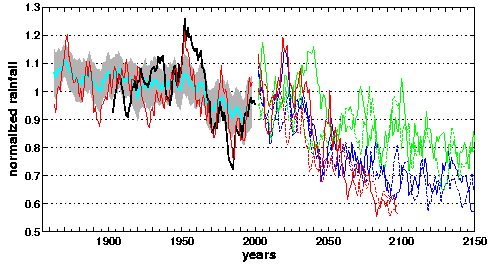
In this figure, the black line is again the observations of summertime Sahel rainfall, but with a 5 year running filter. The red line prior to the year 2000 is a single realization of the freely running coupled model (unconstrained by observed ocean temperatures) but forced with estimates of the evolving greenhouse gas, aerosol, and volcanic forcing since 1860. One does not expect a particular freely running simulation of this type to simulate observations which depend in part on details of the natural variability in the oceans, but, given enough realizations, one does expect to find some that resemble observations. The realization chosen here is the one (out of 6) that most resembles the observed time series. The gray area shows the typical spread of the realizations (plus and minus one standard deviation), while the thick blue line is the mean of this ensemble. The mean provides an estimate of the forced part of the variation, which further analysis indicates is partly due to greenhouse gases in this simulation and partly to aerosol forcing. The three colors of lines continuing into the 21st century are the results of simulations of the future (using the B1(green), A1B(blue), and A2(red) SRES scenarios).
The average over the climate models utilized by the IPCC AR4 assessment is for little change in Sahel rainfall, with a number of models generating modest increases in rainfall, some generated modest drying, but no others producing the dramatic drying in CM2 One is tempted to reject this simulation as an outlier. Analysis supporting this position is provided by Cook and Vizy and Cook .
We are hopeful that we will find serious flaws in our model that would cause us to reject its projection as unrealistic. There are metrics based on comparison with a variety of observations that one can try to use to evaluate climate models. Ideally, there would be an model property A (for which there are observations to compare against) which takes on values in the world’s climate models that are correlated with, or, more generally, can be used to predict, the 21st century Sahel rainfall projections in this same set of models. The difference between a model’s A and the observed A then provides a metric that is demonstrably relevant to Sahel rainfall projection. There are several excellent examples of this approach in the literature, related to other aspects of climate projections, a particularly clean example being the study of snow albedo feedback by Hall and Qu . An informative but unsuccessful attempt to isolate such a metric from the co-variability of ocean temperatures and Sahel rainfall in the models is described by Biasutti, Held, Sobel, and Gianinni . In the absence of an ability to predict a model’s Sahel rainfall projection from a model’s behavior in the 20th century, we are left with subjective metrics that are not fully satisfying.
Plausible sources of this difference between AM2/LM2 and other models can be found in the land surface model and in the treatment of convection and clouds in the atmosphere. Our analysis indicates that it is the latter, convection and clouds, that are the most likely source of our model’s outlier status — specifically, we can change the atmospheric model’s response over the Sahel to a uniform warming of ocean temperatures by modifying the treatment of convection/clouds, but have not found comparable sensitivity to the land model. While we believe we can modify our model to generate much weaker drying in its Sahel projections, we have not yet been able to generate a model of this type that has a 20th century Sahel rainfall simulation of comparable quality.
Therefore, we are not yet ready to reject the hypothesis that, superposed on large multi-decadal internal climate variations, and on aerosol induced changes, there is also a suggestion of a greenhouse induced drying trend that will emerge clearly over the present century. More detailed information on internal variability in the Sahel over longer time scales is of vital importance, as is a much clearer understanding of why climate models differ even in the sign of the change expected in Sahel rainfall from increasing greenhouse gases.
prepared by Isaac Hel d for the purpose of placing GFDL research on this subject into a larger context — this essay should not be thought of as a summary of all relevant research on this issue
Comments welcome — isaac.held at noaa.gov.
Thank you for visiting nature.com. You are using a browser version with limited support for CSS. To obtain the best experience, we recommend you use a more up to date browser (or turn off compatibility mode in Internet Explorer). In the meantime, to ensure continued support, we are displaying the site without styles and JavaScript.
- View all journals
- Explore content
- About the journal
- Publish with us
- Sign up for alerts
- News & Views
- Published: October 2008
Climate science
The mysteries of Sahel droughts
- Kerry H. Cook 1
Nature Geoscience volume 1 , pages 647–648 ( 2008 ) Cite this article
1143 Accesses
64 Citations
6 Altmetric
Metrics details
Past variability in Sahel rainfall is closely linked to global sea surface temperature distributions in observations and models alike. Climate simulations for the 21st century suggest that additional influences may become important in the future.
This is a preview of subscription content, access via your institution
Relevant articles
Open Access articles citing this article.
Resolution dependence of CO2-induced Tropical Atlantic sector climate changes
- & M. Latif
npj Climate and Atmospheric Science Open Access 15 September 2020
The Lake Chad hydrology under current climate change
- Binh Pham-Duc
- , Florence Sylvestre
- … Jean-Francois Crétaux
Scientific Reports Open Access 26 March 2020
Sahel rainfall strength and onset improvements due to more realistic Atlantic cold tongue development in a climate model
- , J. Harlaß
- … M. Latif
Scientific Reports Open Access 07 February 2018
Access options
Subscribe to this journal
Receive 12 print issues and online access
251,40 € per year
only 20,95 € per issue
Buy this article
- Purchase on Springer Link
- Instant access to full article PDF
Prices may be subject to local taxes which are calculated during checkout
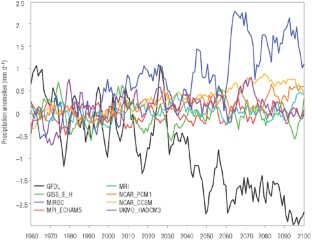
Dilley, J., Chen, R. S., Deichmann, U., Lerner-Lam, A. L. & Arnold, M. Natural Disaster Hotspots: A Global Risk Analysis (The World Bank, 2005).
Book Google Scholar
Charney, J., Stone, P. H., Quirk, W. J. Science 187 , 434–435 (1975).
Article Google Scholar
Folland, C. K., Palmer, T. N., Parker, D. E. Nature 3210 , 602–607 (1986).
Rowell, D. P. Quart. J. Roy. Meteor. Soc. 127 , 1683–1706 (2001).
Hoerling, M., Hurrell, J., Eischeid, J. & Phillips, A. J. Climate 19 , 3989–4008 (2006).
Paeth, H. & Hense, A. Climatic Change 65 , 179–208 (2006).
Hagos, S. M. & Cook, K. H. J. Climate 21 , 3797–3814 (2008).
Biasutti, M., Held, I. M., Sobel, A. H. & Giannini, A. J Climate , 21 , 3471–3486 (2008).
Solomon, S. et al. (eds) Climate Change 2007: The Physical Science Basis — Contribution of Working Group 1 to the Fourth Assessment Report of the Intergovernmental Panel on Climate Change (Cambridge Univ. Press, Cambridge, UK, 2007).
Google Scholar
Joly, M., Voldoire, A., Douville, H., Terray, P. & Royer, J. F. Climate Dynamics 29 , 1–20 (2007).
Cook, K. H. & Vizy, E. K. J. Climate 19 , 3681–3703 (2006).
Koster, R. D. et al. J. Hydrometeor. 7 , 590–610 (2006).
Download references
Author information
Authors and affiliations.
Kerry H. Cook is at the Jackson School of Geosciences, University of Texas at Austin, 1 University Station C1100, Austin, Texas 78712, USA. [email protected],
Kerry H. Cook
You can also search for this author in PubMed Google Scholar
Rights and permissions
Reprints and permissions
About this article
Cite this article.
Cook, K. The mysteries of Sahel droughts. Nature Geosci 1 , 647–648 (2008). https://doi.org/10.1038/ngeo320
Download citation
Issue Date : October 2008
DOI : https://doi.org/10.1038/ngeo320
Share this article
Anyone you share the following link with will be able to read this content:
Sorry, a shareable link is not currently available for this article.
Provided by the Springer Nature SharedIt content-sharing initiative
This article is cited by
Review of meteorological drought in africa: historical trends, impacts, mitigation measures, and prospects.
- Brian Ayugi
- Emmanuel Olaoluwa Eresanya
- Victor Ongoma
Pure and Applied Geophysics (2022)
npj Climate and Atmospheric Science (2020)
- Florence Sylvestre
- Jean-Francois Crétaux
Scientific Reports (2020)
Projected changes in the terrestrial and oceanic regulators of climate variability across sub-Saharan Africa
- Michael Notaro
Climate Dynamics (2020)
Projection of vegetation impacts on future droughts over West Africa using a coupled RegCM-CLM-CN-DV
- Muhammad Shafqat Mehboob
- Yeonjoo Kim
- Guiling Wang
Climatic Change (2020)
Quick links
- Explore articles by subject
- Guide to authors
- Editorial policies
Sign up for the Nature Briefing newsletter — what matters in science, free to your inbox daily.

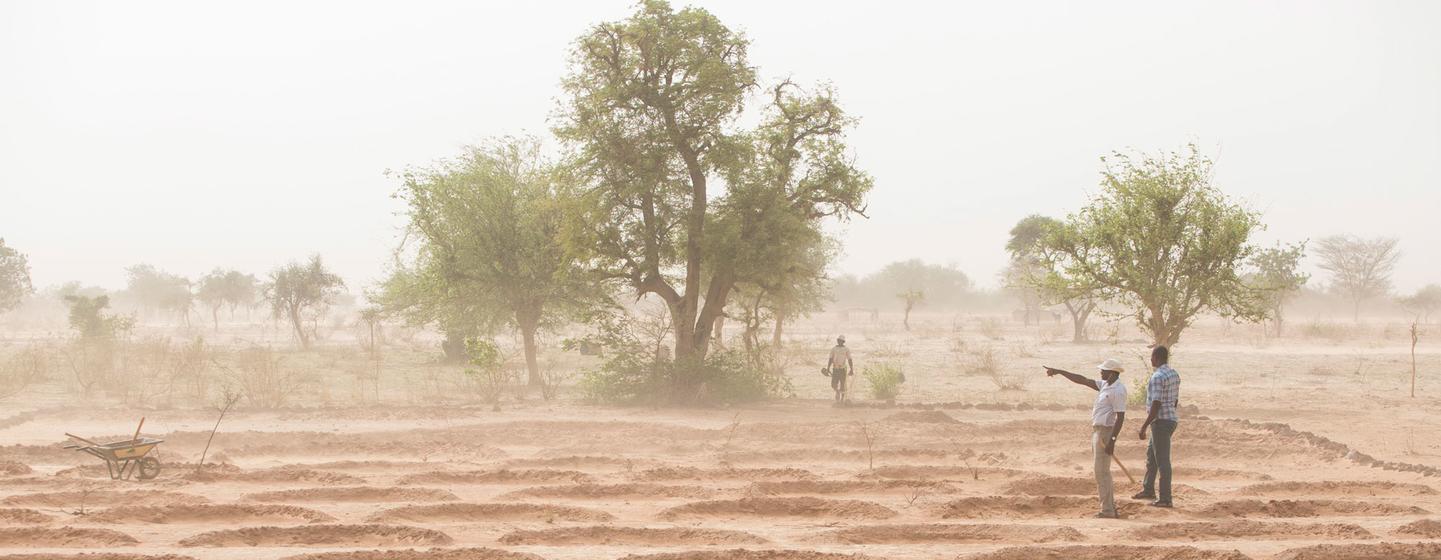
Bringing dry land in the Sahel back to life
Facebook Twitter Print Email
Millions of hectares of farmland are lost to the desert each year in Africa’s Sahel region, but the UN Food and Agriculture Organization (FAO) is showing that traditional knowledge, combined with the latest technology, can turn arid ground back into fertile soil.
Those trying to grow crops in the Sahel region are often faced with poor soil, erratic rainfail and long periods of drought. However, the introduction of a state-of-the art heavy digger, the Delfino plough, is proving to be, literally, a breakthrough.
As part of its Action Against Desertification (AAD) programme, the FAO has brought the Delfino to four countries in the Sahel region – Burkina Faso, Niger, Nigeria and Senegal – to cut through impacted, bone-dry soil to a depth of more than half a metre.
The Delfino plough is extremely efficient: one hundred farmers digging irrigation ditches by hand can cover a hectare a day, but when the Delfino is hooked to a tractor, it can cover 15 to 20 hectares in a day.
Once an area is ploughed, the seeds of woody and herbaceous native species are then sown directly, and inoculated seedlings planted. These species are very resilient and work well in degraded land, providing vegetation cover and improving the productivity of previously barren lands.
In Burkina Faso and Niger, the target number of hectares for immediate restoration has already been met and extended thanks to the Delfino plough. In Nigeria and Senegal, it is working to scale up the restoration of degraded land.
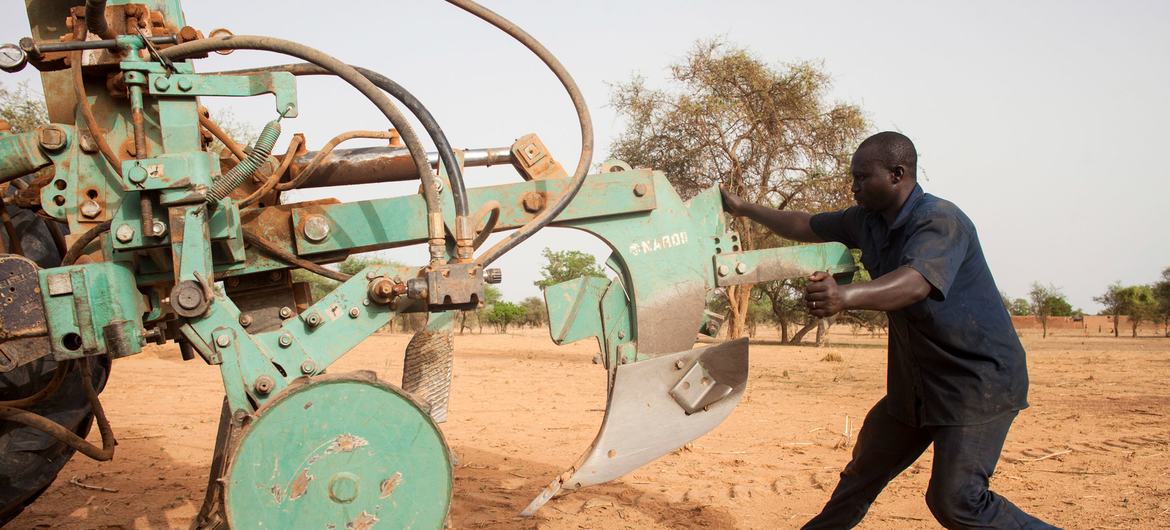
Farming seen through a half-moon lens
This technology, whilst impressive, is proving to be successful because it is being used in tandem with traditional farming techniques.
“In the end the Delfino is just a plough. A very good and suitable plough, but a plough all the same,” says Moctar Sacande, Coordinator of FAO’s Action Against Desertification programme. “It is when we use it appropriately and in consultation and cooperation that we see such progress.”
The half-moon is a traditional Sahel planting method which creates contours to stop rainwater runoff, improving water infiltration and keeping the soil moist for longer. This creates favourable micro-climate conditions allowing seeds and seedlings to flourish.
The Delfino creates large half-moon catchments ready for planting seeds and seedlings, boosting rainwater harvesting tenfold and making soil more permeable for planting than the traditional - and backbreaking – method of digging by hand.
“The whole community is involved and has benefitted from fodder crops such as hay as high as their knees within just two years”, says Mr. Sacande. “They can feed their livestock and sell the surplus, and move on to gathering products such as edible fruits, natural oils for soaps, wild honey and plants for traditional medicine”.
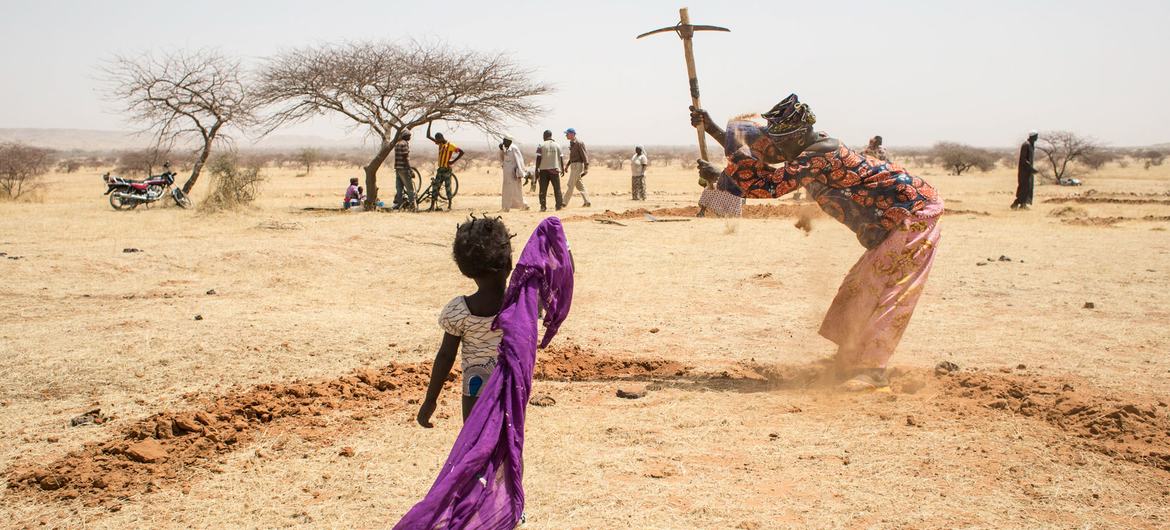
Women taking the lead
According to Nora Berrahmouni, who was FAO’s Senior Forestry Officer for the African Regional Office when the Delfino was deployed, the plough will also reduce the burden on women.
“The season for the very hard work of hand-digging the half-moon irrigation dams comes when the men of the community have had to move with the animals. So, the work falls on the women,” says Ms. Berrahmouni.
Because the Delfino plough significantly speeds up the ploughing process and reduces the physical labour needed, it gives women extra time to manage their multitude of other tasks.
The project also aims to boost women’s participation in local land restoration on a bigger scale, offering them leadership roles through the village committees that plan the work of restoring land. Under the AAD programme, each site selected for restoration is encouraged to set up a village committee to manage the resources, so as to take ownership right from the beginning.
“Many women are running the local village committees which organise these activities and they are telling us they feel more empowered and respected,” offers Mr. Sacande.
Respecting local knowledge and traditional skills is another key to success. Communities have long understood that half-moon dams are the best way of harvesting rainwater for the long dry season. The mighty Delfino is just making the job more efficient and less physically demanding.
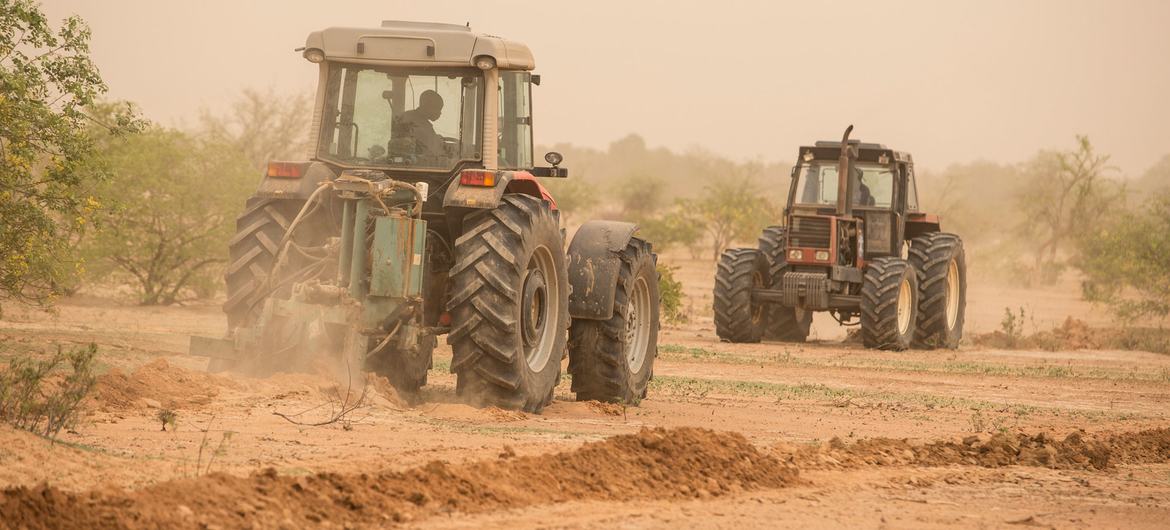
Millions of hectares lost to the desert, forests under threat
And it is urgent that progress is made. Land loss is a driver of many other problems such as hunger, poverty, unemployment, forced migration, conflict and an increased risk of extreme weather events related to climate change.
In Burkina Faso, for example, a third of the landscape is degraded. This means that over nine million hectares of land, once used for agriculture, is no longer viable for farming.
It is projected that degradation will continue to expand at 360 000 hectares per year. If the situation is not reversed, forests are at risk of being cleared to make way for productive agricultural land.
Africa is currently losing four million hectares of forest every year for this reason, yet has more than 700 million hectares of degraded land viable for restoration. By bringing degraded land back to life, farmers do not have to clear additional forest land to turn into cropland for Africa’s rising population and growing food demands.
When Mr. Sacande talks about restoring land in Africa, the passion in his voice is evident. “Restoring degraded land back to productive good health is a huge opportunity for Africa. It brings big social and economic benefits to rural farming communities,” he says. “It’s a bulwark against climate change and it brings technology to enhance traditional knowledge.”
A version of this story first appeared on the FAO website .
- Burkina Faso
- agriculture
- Desertification

- 1. Introduction
- a. Influence of the tropical Atlantic Ocean
- b. Influence of the Mediterranean Sea
- c. Influence of the Pacific Ocean
- d. Influence of the Indian Ocean
- e. Modulation of the interannual variability by lower-frequency variations
- a. Role of SST anomalies
- b. Role of land surface processes
- 4. Seasonal forecasting of drought in Africa
- 5. Decadal prediction of the WAM
- 6. Future projections
- b. Numerical modeling issues
- c. Remaining questions
- d. Future directions
Ackerley , D. , B. Booth , S. H. E. Knight , E. J. Highwood , D. J. Frame , M. R. Allen , and D. P. Rowell , 2011 : Sensitivity of twentieth-century Sahel rainfall to sulfate aerosol and CO 2 forcing . J. Climate , 24 , 4999 – 5014 , doi: 10.1175/JCLI-D-11-00019.1 .
- Search Google Scholar
- Export Citation
AMMA , 2010 : The International Science Plan 2010–2020. African Monsoon Multidisciplinary Analyses, 136 pp. [Available online at www.amma-international.org/IMG/pdf/ISP2_v2.pdf .]
Bader , J. , and M. Latif , 2003 : The impact of decadal scale Indian Ocean SST anomalies on Sahelian rainfall and the North Atlantic Oscillation . Geophys. Res. Lett. , 30 , 2169 , doi: 10.1029/2003GL018426 .
Bader , J. , and M. Latif , 2011 : The 1983 drought in the West Sahel: A case study . Climate Dyn. , 36 , 463 – 472 , doi: 10.1007/s00382-009-0700-y .
Batté , L. , and M. Déqué , 2011 : Seasonal predictions of precipitation over Africa using coupled ocean–atmosphere general circulation models: Skill of the ENSEMBLES project multimodel ensemble forecasts . Tellus , 63A , 283 – 299 , doi: 10.1111/j.1600-0870.2010.00493.x .
Biasutti , M. , 2011 : Atmospheric science: A man-made drought . Nat. Climate Change , 1 , 197 – 198 , doi: 10.1038/nclimate1151 .
Biasutti , M. , 2013 : Forced Sahel rainfall trends in the CMIP5 archive . J. Geophys. Res. , 118 , 1613–1623 , doi: 10.1002/jgrd.50206 .
Biasutti , M. , and A. Giannini , 2006 : Robust Sahel drying in response to late 20th century forcings . Geophys. Res. Lett. , 33 , L11706 , doi: 10.1029/2006GL026067 .
Biasutti , M. , and A. H. Sobel , 2009 : Delayed Sahel rainfall and global seasonal cycle in a warmer climate . Geophys. Res. Lett. , 36 , L23707 , doi: 10.1029/2009GL041303 .
Biasutti , M. , A. H. Sobel , and Y. Kushnir , 2006 : AGCM precipitation biases in the tropical Atlantic . J. Climate , 19 , 935 – 958 , doi: 10.1175/JCLI3673.1 .
Biasutti , M. , I. M. Held , A. H. Sobel , and A. Giannini , 2008 : SST forcings and Sahel rainfall variability in simulations of the twentieth and twenty-first centuries . J. Climate , 21 , 3471 – 3486 , doi: 10.1175/2007JCLI1896.1 .
Boone , A. , I. Poccard-Leclerq , Y. Xue , J. Feng , and P. de Rosnay , 2010 : Evaluation of the WAMME model surface fluxes using results from the AMMA land-surface model intercomparison project . Climate Dyn. , 35 , 127 – 142 , doi: 10.1007/s00382-009-0653-1 .
Booth , B. B. , N. J. Dunstone , P. R. Halloran , T. Andrews , and N. Bellouin , 2012 : Aerosols implicated as a prime driver of twentieth-century North Atlantic climate variability . Nature , 484 , 228 – 232 , doi: 10.1038/nature10946 .
Brooks , G. E. , 1998 : Climate and history in West Africa. Transformations in Africa: Essays on Africa’s Later Past, G. Connah, Ed., University Press, 139–159 .
Camberlin , P. , S. Janicot , and I. Poccard , 2001 : Seasonality and atmospheric dynamics of the teleconnection between African rainfall and tropical sea-surface temperature: Atlantic vs. ENSO . Int. J. Climatol. , 21 , 973 – 1005 , doi: 10.1002/joc.673 .
Caminade , C. , and L. Terray , 2010 : Twentieth century Sahel rainfall variability as simulated by the ARPEGE AGCM, and future changes . Climate Dyn. , 35 , 75 – 94 , doi: 10.1007/s00382-009-0545-4 .
Caminade , C. , and Coauthors , 2011 : Mapping Rift Valley fever and malaria risk over West Africa using climatic indicators . Atmos. Sci. Lett. , 12 , 96 – 103 , doi: 10.1002/asl.296 .
Caminade , C. , and Coauthors , 2014 : Impact of climate change on global malaria distribution. Proc. Natl. Acad. Sci. USA, 111, 3286–3291 , doi: 10.1073/pnas.1302089111 .
Carton , J. A. , X. Cao , B. S. Giese , and A. M. Da Silva , 1996 : Decadal and interannual SST variability in the tropical Atlantic . Ocean. J. Phys. Oceanogr. , 26 , 1165 – 1175 , doi: 10.1175/1520-0485(1996)026<1165:DAISVI>2.0.CO;2 .
Cash , B. A. , X. Rodó , J. Ballester , M. J. Bouma , A. Baeza , R. Dhiman , and M. Pascual , 2013 : Malaria epidemics and the influence of the tropical South Atlantic on the Indian monsoon . Nat. Climate Change , 3 , 502 – 507 , doi: 10.1038/nclimate1834 .
Charney , J. G. , 1975 : Dynamics of deserts and drought in the Sahel . Quart. J. Roy. Meteor. Soc. , 101 , 193 – 202 , doi: 10.1002/qj.49710142802 .
Chiang , J. C. H. , and A. H. Sobel , 2002 : Tropical tropospheric temperature variations caused by ENSO and their influence on the remote tropical climate . J. Climate , 15 , 2616 – 2631 , doi: 10.1175/1520-0442(2002)015<2616:TTTVCB>2.0.CO;2 .
Chin , M. , and Coauthors , 2014 : Multi-decadal aerosol variations from 1980 to 2009: A perspective from observations and a global model . Atmos. Chem. Phys. , 14 , 3657 – 3690 , doi: 10.5194/acp-14-3657-2014 .
Chou , C. , and C.-W. Lan , 2012 : Changes in the annual range of precipitation under global warming . J. Climate , 25 , 222 – 235 , doi: 10.1175/JCLI-D-11-00097.1 .
Chou , C. , J. C. H. Chiang , C.-W. Lan , C.-H. Chung , Y.-C. Liao , and C.-J. Lee , 2013 : Increase in the range between wet and dry season precipitation . Nat. Geosci. , 6 , 263 – 267 , doi: 10.1038/ngeo1744 .
Cook , K. H. , 2008 : The mysteries of Sahel droughts . Nat. Geosci. , 1 , 647 – 648 , doi: 10.1038/ngeo320 .
Davey , M. K. , and Coauthors , 2001 : STOIC: A study of coupled model climatology and variability in tropical ocean regions . Climate Dyn. , 18 , 403 – 420 , doi: 10.1007/s00382-001-0188-6 .
Dezfuli , A. K. , and S. E. Nicholson , 2011 : A note on long-term variations of the African easterly jet . Int. J. Climatol. , 31 , 2049 – 2054 , doi: 10.1002/joc.2209 .
Doblas-Reyes , F. J. , A. Weisheimer , T. N. Palmer , J. M. Murphy , and D. Smith , 2010 : Forecast quality assessment of the ENSEMBLES seasonal-to-decadal Stream 2 hindcasts. ECMWF Tech. Memo. 621, 45 pp .
Doblas-Reyes , F. J. , and Coauthors , 2013 : Initialized near-term regional climate change prediction. Nature Commun., 4, 1715 , doi: 10.1038/ncomms2704 .
Douville , H. , 2002 : Influence of soil moisture on the Asian and African monsoon. Part II: Interannual variability . J. Climate , 15 , 701 – 720 , doi: 10.1175/1520-0442(2002)015<0701:IOSMOT>2.0.CO;2 .
Douville , H. , S. Conil , S. Tyteca , and A. Voldoire , 2007 : Soil moisture memory and West African monsoon predictability: Artefact or reality? Climate Dyn. , 28 , 723 – 742 , doi: 10.1007/s00382-006-0207-8 .
Druyan , L. M. , 2011 : Studies of 21st-century precipitation trends over West Africa . Int. J. Climatol. , 31 , 1415 – 1424 , doi: 10.1002/joc.2180 .
Druyan , L. M. , and Coauthors , 2010 : The WAMME regional model intercomparison study . Climate Dyn. , 35 , 175 – 192 , doi: 10.1007/s00382-009-0676-7 .
Eltahir , E. A. B. , and C. Gong , 1996 : Dynamics of wet and dry years in West Africa . J. Climate , 9 , 1030 – 1042 , doi: 10.1175/1520-0442(1996)009<1030:DOWADY>2.0.CO;2 .
Ermert , V. , A. H. Fink , and H. Paeth , 2013 : The potential effects of climate change on malaria transmission in Africa using bias-corrected regionalised climate projections and a simple malaria seasonality model . Climatic Change , 120 , 741 – 754 , doi: 10.1007/s10584-013-0851-z .
Folland , C. K. , T. N. Palmer , and D. E. Parker , 1986 : Sahel rainfall and worldwide sea temperatures, 1901–85 . Nature , 320 , 602 – 607 , doi: 10.1038/320602a0 .
Fontaine , B. , and S. Janicot , 1996 : Sea surface temperature fields associated with West African rainfall anomaly types . J. Climate , 9 , 2935 – 2940 , doi: 10.1175/1520-0442(1996)009<2935:SSTFAW>2.0.CO;2 .
Fontaine , B. , and Coauthors , 2010 : Impacts of warm and cold situations in the Mediterranean basins on the West African monsoon: Observed connection patterns (1979–2006) and climate simulations . Climate Dyn. , 35 , 95 – 114 , doi: 10.1007/s00382-009-0599-3 .
Fontaine , B. , M. Gaetani , A. Ullmann , and P. Roucou , 2011a : Time evolution of observed July–September sea surface temperature-Sahel climate teleconnection with removed quasi-global effect (1900–2008). J. Geophys. Res., 116, D04105 , doi: 10.1029/2010JD014843 .
Fontaine , B. , P.-A. Monerie , M. Gaetani , and P. Roucou , 2011b : Climate adjustments over the African-Indian monsoon regions accompanying Mediterranean Sea thermal variability. J. Geophys. Res., 116, D23122 , doi: 10.1029/2011JD016273 .
Frich , P. , L. V. Alexander , P. Della-Marta , B. Gleason , M. Haylock , A. M. G. Klein Tank , and T. Peterson , 2002 : Observed coherent changes in climatic extremes during the second half of the twentieth century . Climate Res. , 19 , 193 – 212 , doi: 10.3354/cr019193 .
Gaetani , M. , and E. Mohino , 2013 : Decadal prediction of the Sahelian precipitation in CMIP5 simulations . J. Climate , 26 , 7708 – 7719 , doi: 10.1175/JCLI-D-12-00635.1 .
Gaetani , M. , B. Fontaine , P. Roucou , and M. Baldi , 2010 : Influence of the Mediterranean Sea on the West African monsoon: Intraseasonal variability in numerical simulations . J. Geophys. Res. , 115 , D24115 , doi: 10.1029/2010JD014436 .
García-Serrano , J. , F. J. Doblas-Reyes , R. J. Haarsma , and I. Polo , 2013 : Decadal prediction of the dominant West African monsoon rainfall modes . J. Geophys. Res. , 118 , 5260 – 5279 , doi: 10.1002/jgrd.50465 .
García-Serrano , J. , V. Guemas , and F. J. Doblas-Reyes , 2015 : Added-value from initialization in predictions of Atlantic multi-decadal variability . Climate Dyn. , doi: 10.1007/s00382-014-2370-7 , in press .
Giannini , A. , 2010 : Mechanisms of climate change in the semi-arid African Sahel: The local view . J. Climate , 23 , 743 – 756 , doi: 10.1175/2009JCLI3123.1 .
Giannini , A. , R. Saravanan , and P. Chang , 2003 : Oceanic forcing of Sahel rainfall on interannual to interdecadal time scales . Science , 302 , 1027 – 1030 , doi: 10.1126/science.1089357 .
Giannini , A. , S. Salack , T. Lodoun , A. Ali , A. T. Gaye , and O. Ndiaye , 2013 : A unifying view of climate change in the Sahel linking intra-seasonal, interannual and longer time scales . Environ. Res. Lett. , 8 , 024010 , doi: 10.1088/1748-9326/8/2/024010 .
Goddard , L. , and Coauthors , 2010 : Providing seasonal-to-interannual climate information for risk management and decision-making . Proc. Environ. Sci. , 1 , 81 – 101 , doi: 10.1016/j.proenv.2010.09.007 .
Goddard , L. , and Coauthors , 2013 : A verification framework for interannual-to-decadal prediction experiments . Climate Dyn. , 40 , 245 – 272 , doi: 10.1007/s00382-012-1481-2 .
Goutorbe , J. P. , and Coauthors , 1997 : An overview of HAPEX-Sahel: A study in climate and desertification . J. Hydrol. , 188–189 , 4 – 17 , doi: 10.1016/S0022-1694(96)03308-2 .
Graham , R. J. , and Coauthors , 2011 : Long-range forecasting and the Global Framework for Climate Services . Climate Res. , 47 , 47–55 , doi: 10.3354/cr00963 .
Gu , G. , and R. F. Adler , 2006 : Interannual rainfall variability in the tropical Atlantic region . J. Geophys. Res. , 111 , D02106 , doi: 10.1029/2005JD005944 .
Guemas , V. , J. García-Serrano , A. Mariotti , F. J. Doblas-Reyes , and L.-P. Caron , 2015 : Prospects for decadal climate prediction in the Mediterranean region . Quart. J. Roy. Meteor. Soc. , doi: 10.1002/qj.2379 , in press .
Haarsma , R. J. , F. M. Selten , S. L. Weber , and M. Kliphuis , 2005 : Sahel rainfall variability and response to greenhouse warming . Geophys. Res. Lett. , 32 , L17702 , doi: 10.1029/2005GL023232 .
Hagos , S. , and K. Cook , 2008 : Ocean warming and late-twentieth-century Sahel drought and recovery . J. Climate , 21 , 3797 – 3814 , doi: 10.1175/2008JCLI2055.1 .
Harris , I. , P. D. Jones , T. J. Osborn , and D. H. Lister , 2014 : Updated high-resolution grids of monthly climatic observations—The CRU TS3.10 Dataset . Int. J. Climatol. , 34 , 623 – 642 , doi: 10.1002/joc.3711 .
Hastenrath , S. , 1984 : Interannual variability and annual cycle: Mechanisms of circulation and climate in the tropical Atlantic sector . Mon. Wea. Rev. , 112 , 1097 – 1107 , doi: 10.1175/1520-0493(1984)112<1097:IVAACM>2.0.CO;2 .
Hastenrath , S. , and P. J. Lamb , 1977 : Some aspects of circulation and climate over the eastern equatorial Atlantic . Mon. Wea. Rev. , 105 , 1019 – 1023 , doi: 10.1175/1520-0493(1977)105<1019:SAOCAC>2.0.CO;2 .
Hastenrath , S. , and D. Polzin , 2011 : Long-term variations of circulation in the tropical Atlantic sector and Sahel rainfall . Int. J. Climatol. , 31 , 649 – 655 , doi: 10.1002/joc.2116 .
Haywood , J. M. , A. Jones , N. Bellouin , and D. Stephenson , 2013 : Asymmetric forcing from stratospheric aerosols impacts Sahelian rainfall . Nat. Climate Change , 3 , 660 – 665 , doi: 10.1038/nclimate1857 .
Held , I. M. , and B. J. Soden , 2006 : Robust responses of the hydrological cycle to global warming . J. Climate , 19 , 5686 – 5699 , doi: 10.1175/JCLI3990.1 .
Held , I. M. , T. L. Delworth , J. Lu , K. L. Findell , and T. R. Knutson , 2005 : Simulation of Sahel drought in the 20th and 21st centuries . Proc. Natl. Acad. Sci. USA , 102 , 17 891 – 17 896 , doi: 10.1073/pnas.0509057102 .
Hernández-Díaz , L. , R. Laprise , L. Sushama , A. Martynov , K. Winger , and B. Dugas , 2013 : Climate Dyn. , 40 , 1415 – 1433 , doi: 10.1007/s00382-012-1387-z .
Hewitt , C. D. , 2004 : ENSEMBLES-based predictions of climate changes and their impacts. Eos, Trans. Amer. Geophys. Union, 85, 566 , doi: 10.1029/2004EO520005 .
Hoerling , M. , J. W. Hurrell , J. Eischeid , and A. S. Phillips , 2006 : Detection and attribution of twentieth-century northern and southern African rainfall change . J. Climate , 19 , 3989 – 4008 , doi: 10.1175/JCLI3842.1 .
Hoerling , M. , J. Eisheid , and J. Perlwitz , 2010 : Regional precipitation trends: Distinguishing natural variability from anthropogenic forcing . J. Climate , 23 , 2131 – 2145 , doi: 10.1175/2009JCLI3420.1 .
Horel , J. D. , V. E. Kousky , and M. T. Kagano , 1986 : Atmospheric conditions in the Atlantic sector during 1983 and 1984 . Nature , 322 , 248 – 251 , doi: 10.1038/322248a0 .
Hoshen , M. B. , and A. P. Morse , 2004 : A weather-driven model of malaria transmission . Malar. J. , 3 , 32 , doi: 10.1186/1475-2875-3-32 .
Huffman , G. J. , and Coauthors , 2007 : The TRMM Multi-satellite Precipitation Analysis: Quasi-global, multi-year, combined-sensor precipitation estimates at fine scale . J. Hydrometeor. , 8 , 38 – 55 , doi: 10.1175/JHM560.1 .
Hwang , Y.-T. , D. M. W. Frierson , and S. M. Kang , 2013 : Anthropogenic sulfate aerosol and the southward shift of tropical precipitation in the late 20th century . Geophys. Res. Lett. , 40 , 2845 – 2850 , doi: 10.1002/grl.50502 .
Ingram , K. T. , M. C. Roncoli , and P. H. Kirshen , 2002 : Opportunities and constraints for farmers of West Africa to use seasonal precipitation forecasts with Burkina Faso as a case study . Agric. Syst. , 74 , 331 – 349 , doi: 10.1016/S0308-521X(02)00044-6 .
Janicot , S. , V. Moron , and B. Fontaine , 1996 : ENSO dynamics and Sahel droughts . Geophys. Res. Lett. , 23 , 515 – 518 , doi: 10.1029/96GL00246 .
Janicot , S. , A. Harzallah , B. Fontaine , and V. Moron , 1998 : West African monsoon dynamics and eastern equatorial Atlantic and Pacific SST anomalies (1970–88) . J. Climate , 11 , 1874 – 1882 , doi: 10.1175/1520-0442-11.8.1874 .
Janicot , S. , S. Trzaska , and I. Poccard , 2001 : Summer Sahel–ENSO teleconnection and decadal time scale SST variations . Climate Dyn. , 18 , 303 – 320 , doi: 10.1007/s003820100172 .
Joly , M. , and A. Voldoire , 2009 : Influence of ENSO on the West African monsoon: Temporal aspects and atmospheric processes . J. Climate , 22 , 3193 – 3210 , doi: 10.1175/2008JCLI2450.1 .
Joly , M. , and A. Voldoire , 2010 : Role of the Gulf of Guinea in the inter-annual variability of the West African monsoon: What do we learn from CMIP3 coupled simulations? Int. J. Climatol. , 30 , 1843 – 1856 .
Joly , M. , A. Voldoire , H. Douville , P. Terray , and J. F. Royer , 2007 : African monsoon teleconnections with tropical SSTs: Validation and evolution in a set of IPCC4 simulations . Climate Dyn. , 29, 1–20 , doi: 10.1007/s00382-006-0215-8 .
Jung , T. , L. Ferranti , and A. M. Tompkins , 2006 : Response to the summer of 2003 Mediterranean SST anomalies over Europe and Africa (2006) . J. Climate , 19 , 5439 – 5454 , doi: 10.1175/JCLI3916.1 .
Kang , H.-S. , Y. Xue , and G. J. Collatz , 2007 : Assessment of satellite-derived leaf area index datasets using a general circulation model: Seasonal variability . J. Climate , 20 , 993 – 1015 , doi: 10.1175/JCLI4054.1 .
Kang , S. M. , D. M. W. Frierson , and I. M. Held , 2009 : The tropical response to extratropical thermal forcing in an idealized GCM: The importance of radiative feedbacks and convective parameterization . J. Atmos. Sci. , 66 , 2812 – 2827 , doi: 10.1175/2009JAS2924.1 .
Kawase , H. , M. Abe , Y. Yamada , T. Takemura , T. Yokohata , and T. Nozawa , 2010 : Physical mechanism of long-term drying trend over tropical North Africa . Geophys. Res. Lett. , 37 , L09706 , doi: 10.1029/2010GL043038 .
Kiladis , G. N. , and H. F. Diaz , 1989 : Global climatic anomalies associated with extremes in the Southern Oscillation . J. Climate , 2 , 1069 – 1090 , doi: 10.1175/1520-0442(1989)002<1069:GCAAWE>2.0.CO;2 .
Kim , K.-M. , W. K.-M. Lau , Y. C. Sud , and G. K. Walker , 2010 : Influence of aerosol-radiative forcings on the diurnal and seasonal cycles of rainfall over West Africa and eastern Atlantic Ocean using GCM simulations . Climate Dyn. , 35 , 115 – 126 , doi: 10.1007/s00382-010-0750-1 .
Knight , J. R. , 2009 : The Atlantic multidecadal oscillation inferred from the forced climate response in coupled general circulation models . J. Climate , 22 , 1610 – 1625 , doi: 10.1175/2008JCLI2628.1 .
Knight , J. R. , R. J. Allan , C. K. Folland , M. Vellinga , and M. E. Mann , 2005 : A signature of persistent natural thermohaline circulation cycles in observed climate . Geophys. Res. Lett. , 32 , L20708 , doi: 10.1029/2005GL024233 .
Knight , J. R. , C. K. Folland , and A. A. Scaife , 2006 : Climate impacts of the Atlantic multidecadal oscillation . Geophys. Res. Lett. , 33 , L17706 , doi: 10.1029/2006GL026242 .
Koster , R. D. , and Coauthors , 2006 : GLACE: The Global Land–Atmosphere Coupling Experiment. Part I: Overview . J. Hydrometeor. , 7 , 590 – 610 , doi: 10.1175/JHM510.1 .
Kucharski , F. , N. Zeng , and E. Kalnay , 2013 : A further assessment of vegetation feedback on decadal Sahel rainfall variability . Climate Dyn. , 40 , 1453 – 1466 , doi: 10.1007/s00382-012-1397-x .
Lamb , P. J. , 1978 : Large-scale tropical Atlantic surface circulation patterns associated with sub-Saharan weather anomalies . Tellus , 30 , 240 – 251 , doi: 10.1111/j.2153-3490.1978.tb00839.x .
Lau , K. M. , and K. M. Kim , 2007 : Cooling of the Atlantic by Saharan dust . Geophys. Res. Lett. , 34 , L23811 , doi: 10.1029/2007GL031538 .
Lau , K. M. , S. S. P. Shen , K.-M. Kim , and H. Wang , 2006 : A multimodel study of the twentieth-century simulations of Sahel drought from the 1970s to 1990s . J. Geophys. Res. , 111 , D07111 , doi: 10.1029/2005JD006281 .
Laval , K. , and L. Picon , 1986 : Effect of a change of the surface albedo of the Sahel on climate . J. Atmos. Sci. , 43 , 2418–2429 , doi: 10.1175/1520-0469(1986)043<2418:EOACOT>2.0.CO;2 .
Le Barbe , L. L. , T. Lebel , and D. Tapsoba , 2002 : Rainfall variability in West Africa during the years 1950–90 . J. Climate , 15 , 187 – 202 , doi: 10.1175/1520-0442(2002)015<0187:RVIWAD>2.0.CO;2 .
Li , W.-P. , Y. Xue , and I. Poccard , 2007 : Numerical investigation of the impact of vegetation indices on the variability of West African summer monsoon . J. Meteor. Soc. Japan , 85A , 363 – 383 , doi: 10.2151/jmsj.85A.363 .
Losada , T. , B. Rodríguez-Fonseca , S. Janicot , S. Gervois , F. Chauvin , and P. Ruti , 2010 : A multi-model approach to the Atlantic equatorial mode: Impact on the West African monsoon . Climate Dyn. , 35 , 29 – 43 , doi: 10.1007/s00382-009-0625-5 .
Losada , T. , B. Rodríguez-Fonseca , E. Mohino , J. Bader , S. Janicot , and C. R. Mechoso , 2012 : Tropical SST and Sahel rainfall: A non-stationary relationship . Geophys. Res. Lett. , 39 , L12705 , doi: 10.1029/2012GL052423 .
Lu , J. , 2009 : The dynamics of the Indian Ocean sea surface temperature forcing of Sahel drought . Climate Dyn. , 33 , 445 – 460 , doi: 10.1007/s00382-009-0596-6 .
Lu , J. , and T. L. Delworth , 2005 : Oceanic forcing of the late 20th century Sahel drought . Geophys. Res. Lett. , 32 , L22706 , doi: 10.1029/2005GL023316 .
Ma , H. , H. Xiao , C. Mechoso , and Y. Xue , 2013 : Sensitivity of global tropical climate to land surface processes: Mean state and interannual variability . J. Climate , 26, 1818–1837 , doi: 10.1175/JCLI-D-12-00142.1 .
MacLeod , D. A. , C. Caminade , and A. P. Morse , 2012 : Useful decadal climate prediction at regional scales? A look at the ENSEMBLES stream 2 decadal hindcasts. Environ. Res. Lett., 7, 044012 , doi: 10.1088/1748-9326/7/4/044012 .
Mahajan , S. , K. J. Evans , J. E. Truesdale , J. J. Hack , and J.-L. Lamarque , 2012 : Interannual tropospheric aerosol variability in the late twentieth century and its impact on tropical Atlantic and West African climate by direct and semidirect effects . J. Climate , 25 , 8031 – 5056 , doi: 10.1175/JCLI-D-12-00029.1 .
Mantua , N. J. , S. R. Hare , Y. Zhang , J. M. Wallace , and R. C. Francis , 1997 : A Pacific interdecadal climate oscillation with impacts on salmon production . Bull. Amer. Meteor. Soc. , 78 , 1069 – 1079 , doi: 10.1175/1520-0477(1997)078<1069:APICOW>2.0.CO;2 .
Marteau , R. , B. Sultan , V. Moron , A. Alhassane , C. Baron , and S. B. Traoré , 2011 : The onset of the rainy season and farmers’ sowing strategy for pearl millet cultivation in southwest Niger . Agric. For. Meteor. , 151 , 1356 – 1369 , doi: 10.1016/j.agrformet.2011.05.018 .
Martin , E. R. , and C. D. Thorncroft , 2014a : The impact of the AMO on the West African monsoon annual cycle. Quart. J. Roy. Meteor. Soc., 140, 31–46 , doi: 10.1002/qj.2107 .
Martin , E. R. , and C. D. Thorncroft , 2014b : Sahel rainfall in multimodel CMIP5 decadal hindcasts . Geophys. Res. Lett. , 41 , 2169 – 2175 , doi: 10.1002/2014GL059338 .
Martin , E. R. , C. D. Thorncroft , and B. B. B. Booth , 2014 : The multidecadal Atlantic SST–Sahel rainfall teleconnection in CMIP5 simulations . J. Climate , 27 , 784 – 806 , doi: 10.1175/JCLI-D-13-00242.1 .
Mechoso , C. R. , and Coauthors , 1995 : The seasonal cycle over the tropical Pacific in coupled ocean–atmosphere general circulation models . Mon. Wea. Rev. , 123 , 2825 – 2838 , doi: 10.1175/1520-0493(1995)123<2825:TSCOTT>2.0.CO;2 .
Meehl , G. A. , and Coauthors , 2009 : Decadal prediction: Can it be skillful? Bull. Amer. Meteor. Soc. , 90 , 1467 – 1485 , doi: 10.1175/2009BAMS2778.1 .
Meehl , G. A. , and Coauthors , 2014 : Decadal climate prediction: An update from the trenches . Bull. Amer. Meteor. Soc. , 95 , 243 – 267 , doi: 10.1175/BAMS-D-12-00241.1 .
Mohino , E. , S. Janicot , and J. Bader , 2011a : Sahelian rainfall and decadal to multidecadal SST variability . Climate Dyn. , 37 , 419 – 440 , doi: 10.1007/s00382-010-0867-2 .
Mohino , E. , B. Rodríguez-Fonseca , T. Losada , S. Gervois , S. Janicot , J. Bader , P. Ruti , and F. Chauvin , 2011b : Changes in the interannual SST-forced signals on West African rainfall: AGCM intercomparison . Climate Dyn. , 37 , 1707 – 1725 , doi: 10.1007/s00382-011-1093-2 .
Mohino , E. , B. Rodríguez-Fonseca , C. R. Mechoso , S. Gervois , P. Ruti , and F. Chauvin , 2011c : Impacts of the tropical Pacific/Indian Oceans on the seasonal cycle of the West African monsoon . J. Climate , 24 , 3878 – 3891 , doi: 10.1175/2011JCLI3988.1 .
Monerie , P. A. , P. Roucou , and B. Fontaine , 2013 : Mid-century effects of climate change on African monsoon dynamics using the A1B emission scenario . Int. J. Climatol. , 33 , 881 – 896 , doi: 10.1002/joc.3476 .
Murphy , J. , and Coauthors , 2010 : Towards prediction of decadal climate variability and change . Procedia Environ. Sci. , 1 , 287 – 304 , doi: 10.1016/j.proenv.2010.09.018 .
Ndiaye , O. , W. M. Neil , and W. M. Thiaw , 2011 : Predictability of seasonal Sahel rainfall using GCMs and lead-time improvements through the use of a coupled model. J. Climate, 24, 1931–1949 , doi: 10.1175/2010JCLI3557.1 .
Neelin , J. D. , C. Chou , and H. Su , 2003 : Tropical drought regions in global warming and El Niño teleconnections . Geophys. Res. Lett. , 30 , 2275 , doi: 10.1029/2003GL018625 .
Nicholson , S. E. , 1978 : Climatic variations in the Sahel and other African regions during the past five centuries . J. Arid Environ. , 1 , 3 – 24 .
Nicholson , S. E. , 1979 : The methodology of historical climate reconstruction and its application to Africa . J. Afr. Hist. , 20 , 31 – 49 , doi: 10.1017/S0021853700016704 .
Nicholson , S. E. , 2000 : Land surface processes and Sahel climate . Rev. Geophys. , 38 , 117 – 139 , doi: 10.1029/1999RG900014 .
Nicholson , S. E. , 2013 : The West African Sahel: A review of recent studies on the rainfall regime and its interannual variability . ISRN Meteor. , 2013 , 43521 , doi: 10.1155/2013/453521 .
Nicholson , S. E. , A. K. Dezfuli , and D. Klotter , 2012 : A two-century precipitation dataset for the continent of Africa . Bull. Amer. Meteor. Soc. , 93 , 1219 – 1231 , doi: 10.1175/BAMS-D-11-00212.1 .
Nikulin , G. , and Coauthors , 2012 : Precipitation climatology in an ensemble of CORDEX-Africa regional climate simulations . J. Climate , 25 , 6057 – 6078 , doi: 10.1175/JCLI-D-11-00375.1 .
Nnamchi , H. C. , and J. Li , 2011 : Influence of the South Atlantic Ocean dipole on West African summer precipitation . J. Climate , 24 , 1184 – 1197 , doi: 10.1175/2010JCLI3668.1 .
Notaro , M. , 2008 : Statistical identification of global hotspots in soil moisture feedbacks among IPCC AR4 models . J. Geophys. Res. , 113 , D09101 , doi: 10.1029/2007JD00919 .
Paeth , H. , K. Born , R. Girmes , R. Podzun , and D. Jacob , 2009 : Regional climate change in tropical and Sahel due to greenhouse forcing and land use changes . J. Climate , 22 , 114 – 132 , doi: 10.1175/2008JCLI2390.1 .
Palmer , T. N. , 1986 : Influence of the Atlantic, Pacific, and Indian Oceans on Sahel rainfall . Nature , 322 , 251 – 253 , doi: 10.1038/322251a0 .
Patricola , C. M. , and K. H. Cook , 2011 : Sub-Saharan northern African climate at the end of the twenty-first century: Forcing factors and climate change processes . Climate Dyn. , 37 , 1165 – 1188 , doi: 10.1007/s00382-010-0907-y .
Peyrillé , P. , and J. P. Lafore , 2007 : An idealized two-dimensional framework to study the West African monsoon. Part II: Large-scale advection and the diurnal cycle . J. Atmos. Sci. , 64 , 2783 – 2803 , doi: 10.1175/JAS4052.1 .
Peyrillé , P. , J. P. Lafore , and J. L. Redelsperger , 2007 : An idealized two-dimensional framework to study the West African monsoon. Part I: Validation and key controlling factors . J. Atmos. Sci. , 64 , 2765 – 2782 , doi: 10.1175/JAS3919.1 .
Philippon , N. , and B. Fontaine , 2002 : The relationship between the Sahelian and previous 2nd Guinean rainy seasons: a monsoon regulation by soil wetness? Ann. Geophys. , 20 , 575 – 582 , doi: 10.5194/angeo-20-575-2002 .
Philippon , N. , F. J. Doblas-Reyes , and P. M. Ruti , 2010 : Skill, reproducibility and potential predictability of the West African monsoon in coupled GCMS . Climate Dyn. , 35, 53–74, 10.1007/s00382-010-0856-5 .
Polo , I. , B. Rodríguez-Fonseca , T. Losada , and J. García-Serrano , 2008 : Tropical Atlantic variability modes (1979–2002). Part I: Time-evolving SST modes related to West African rainfall . J. Climate , 21 , 6457 – 6475 , doi: 10.1175/2008JCLI2607.1 .
Polo , I. , A. Ullmann , P. Roucou , and B. Fontaine , 2011 : Weather regimes in the Euro-Atlantic and Mediterranean sector and relationship with West African rainfall over the period 1989–2008 from a self-organizing maps approach . J. Climate , 24 , 3423 – 3432 , doi: 10.1175/2011JCLI3622.1 .
Reason , C. J. C. , and M. Rouault , 2006 : Sea surface temperature variability in the tropical southeast Atlantic Ocean and West African rainfall. Geophys. Res. Lett., 33, L21705 , doi: 10.1029/2006GL027145 .
Redelsperger , J.-L. , C. D. Thorncroft , A. Diedhiou , T. Lebel , D. J. Parker , and J. Polcher , 2006 : African Monsoon Multidisciplinary Analysis: An international research project and field campaign . Bull. Amer. Meteor. Soc. , 87 , 1739 – 1746 , doi: 10.1175/BAMS-87-12-1739 .
Richter , I. , S.-P. Xie , S. K. Behera , T. Doi , and Y. Masumoto , 2014 : Equatorial Atlantic variability and its relation to mean state biases in CMIP5 . Climate Dyn. , 42 , 171 – 188 , doi: 10.1007/s00382-012-1624-5 .
Rodríguez-Fonseca , B. , I. Polo , J. Garcia-Serrano , L. Losada , E. Mohino , C. R. Mechoso , and F. Kucharski , 2009 : Is the Atlantic Niño dynamically affecting the Pacific ENSO in recent decades? Geophys. Res. Lett. , 36 , L20705 , doi: 10.1029/2009GL040048 .
Rodríguez-Fonseca , B. , and Coauthors , 2011 : Interannual and decadal SST-forced responses of the West African monsoon . Atmos. Sci. Lett. , 12 , 67 – 74 , doi: 10.1002/asl.308 .
Roehrig , R. , D. Bouniol , F. Guichard , F. Hourdin , and J. L. Redelsperger , 2013 : The present and future of the West African monsoon: A process-oriented assessment of CMIP5 simulations along the AMMA transect . J. Climate , 26 , 6471 – 6505 , doi: 10.1175/JCLI-D-12-00505.1 .
Ropelewski , C. F. , and M. S. Halpert , 1989 : Precipitation patterns associated with the high index phase of the Southern Oscillation . J. Climate , 2 , 268 – 284 , doi: 10.1175/1520-0442(1989)002<0268:PPAWTH>2.0.CO;2 .
Rotstayn , L. D. , and U. Lohmann , 2002 : Tropical rainfall trends and the indirect aerosol effect . J. Climate , 15 , 2103 – 2116 , doi: 10.1175/1520-0442(2002)015<2103:TRTATI>2.0.CO;2 .
Rowell , D. P. , 1996 : Reply to comments by Y. C. Sud and W. K.-M. Lau on ‘Variability of summer rainfall over tropical North Africa (1906–92): Observations and modelling’ by D. P. Rowell, C. K. Folland, K. Maskell and M. N. Ward (April 4, 1995, 121, 669–704): Further analysis of simulated interdecadal and interannual variability of summer rainfall over tropical North Africa . Quart. J. Roy. Meteor. Soc. , 122 , 1007 – 1013 , doi: 10.1002/qj.49712253213 .
Rowell , D. P. , 2001 : Teleconnections between the tropical Pacific and the Sahel . Quart. J. Roy. Meteor. Soc. , 127 , 1683 – 1706 , doi: 10.1002/qj.49712757512 .
Rowell , D. P. , 2003 : The impact of Mediterranean SSTs on the Sahelian rainfall season . J. Climate , 16 , 849 – 862 , doi: 10.1175/1520-0442(2003)016<0849:TIOMSO>2.0.CO;2 .
Rowell , D. P. , 2013 : Simulating SST teleconnections to Africa: What is the state of the art? J. Climate , 26 , 5397 – 5418 , doi: 10.1175/JCLI-D-12-00761.1 .
Rowell , D. P. , C. K. Folland , K. Maskell , J. A. Owen , and M. N. Ward , 1992 : Modelling the influence of global sea surface temperatures on the variability and predictability of seasonal Sahel rainfall . Geophys. Res. Lett. , 19 , 905 – 908 , doi: 10.1029/92GL00939 .
Rowell , D. P. , C. K. Folland , K. Maskell , and M. N. Ward , 1995 : Variability of summer rainfall over tropical North Africa (1906–92): Observations and modelling . Quart. J. Roy. Meteor. Soc. , 121 , 669 – 704 , doi: 10.1002/qj.49712152311 .
Ruiz-Barradas , A. , J. A. Carton , and S. Nigam , 2000 : Structure of interannual-to-decadal climate variability in the tropical Atlantic sector . J. Climate , 13 , 3285 – 3297 , doi: 10.1175/1520-0442(2000)013<3285:SOITDC>2.0.CO;2 .
Ruti , P. , and Coauthors , 2011 : The West African climate system: A review of the AMMA model inter-comparison initiatives . Atmos. Sci. Lett. , 12 , 116 – 122 , doi: 10.1002/asl.305 .
Salack , S. , A. Giannini , M. Diakhaté , A. T. Gaye , and B. Muller , 2014 : Oceanic influence on the sub-seasonal to interannual timing and frequency of extreme dry spells over the West African Sahel . Climate Dyn. , 42 , 189 – 201 , doi: 10.1007/s00382-013-1673-4 .
Scaife , A. , and Coauthors , 2009 : The CLIVAR C20C Project: Selected 20th century climate events . Climate Dyn. , 33 , 603–614 , doi: 10.1007/s00382-008-0451-1 .
Servain , J. , A. J. Busalacchi , M. J. McPhaden , A. D. Moura , G. Reverdin , M. Vianna , and S. E. Zebiak , 1998 : A Pilot Research Moored Array in the Tropical Atlantic (PIRATA) . Bull. Amer. Meteor. Soc. , 79 , 2019 – 2032 , doi: 10.1175/1520-0477(1998)079<2019:APRMAI>2.0.CO;2 .
Seth , A. , S. A. Rauscher , M. Rojas , A. Giannini , and S. J. Camargo , 2011 : Enhanced spring convective barrier for monsoons in a warmer world? Climatic Change , 104 , 403 – 414 , doi: 10.1007/s10584-010-9973-8 .
Seth , A. , S. A. Rauscher , M. Biasutti , A. Giannini , S. J. Camargo , and M. Rojas , 2013 : CMIP5 projected changes in the annual cycle of precipitation in monsoon regions . J. Climate , 26 , 7328 – 7351 , doi: 10.1175/JCLI-D-12-00726.1 .
Shanahan , T. M. , and Coauthors , 2009 : Atlantic forcing of Persistent drought in West Africa . Science , 324 , 377 – 380 , doi: 10.1126/science.1166352 .
Shinoda , M. , and R. Kawamura , 1994 : Tropical rainbelt, circulation, and sea surface temperatures associated with the Sahelian rainfall trend . J. Meteor. Soc. Japan , 72 , 341 – 357 .
Shinoda , M. , and Y. Yamaguchi , 2003 : Influence of soil moisture anomaly on temperature in the Sahel: A comparison between wet and dry decades . J. Hydrometeor. , 4 , 437 – 447 , doi: 10.1175/1525-7541(2003)4<437:IOSMAO>2.0.CO;2 .
Smith , D. M. , A. A. Scaife , and B. P. Kirtman , 2012 : What is the current state of scientific knowledge with regard to seasonal and decadal forecasting? Environ. Res. Lett., 7, 015602 , doi: 10.1088/1748-9326/7/1/015602 .
Sud , Y. C. , and A. Molud , 1988 : A GCM simulation study of the influence of Saharan evapotranspiration and surface-albedo anomalies on July circulation and rainfall . Mon. Wea. Rev. , 116 , 2388 – 2400 , doi: 10.1175/1520-0493(1988)116<2388:AGSSOT>2.0.CO;2 .
Sultan , B. , and S. Janicot , 2003 : The West African monsoon dynamics. Part II: The “preonset” and “onset” of the summer monsoon . J. Climate , 16 , 3407 – 3427 , doi: 10.1175/1520-0442(2003)016<3407:TWAMDP>2.0.CO;2 .
Taylor , C. M. , E. F. Lambin , N. Stephenne , R. J. Harding , and R. L. H. Essery , 2002 : The influence of land use change on climate in the Sahel . J. Climate , 15 , 3615 – 3629 , doi: 10.1175/1520-0442(2002)015<3615:TIOLUC>2.0.CO;2 .
Taylor , K. E. , R. J. Stouffer , and G. A. Meehl , 2012 : An overview of CMIP5 and the experiment design . Bull. Amer. Meteor. Soc. , 93 , 485 – 498 , doi: 10.1175/BAMS-D-11-00094.1 .
Ting , M. , Y. Kushnir , R. Seager , and C. Li , 2009 : Forced and internal 20th century SST trends in the North Atlantic . J. Climate , 22 , 1469 – 1481 , doi: 10.1175/2008JCLI2561.1 .
Ting , M. , Y. Kushnir , R. Seager , and C. Li , 2011 : Robust features of Atlantic multi-decadal variability and its climate impacts . Geophys. Res. Lett. , 38 , L17705 , doi: 10.1029/2011GL048712 .
Tippett , M. K. , 2006 : Filtering of GCM simulated Sahel precipitation . Geophys. Res. Lett. , 33 , L01804 , doi: 10.1029/2005GL024923 .
Tippett , M. K. , and A. Giannini , 2006 : Potentially predicable components of African summer rainfall in an SST-forced GCM simulation . J. Climate , 19 , 3133 – 3144 , doi: 10.1175/JCLI3779.1 .
Tompkins , A. M. , and V. Ermert , 2013 : A regional-scale, high resolution dynamical malaria model that accounts for population density, climate and surface hydrology . Malar. J. , 12 , 65 , doi: 10.1186/1475-2875-12-65 .
Traore , A. K. , 2011 : Etude and modélisation de l’influence des processus couplés surface-atmosphere sur la variabilité des pluies et du climat ouest africain. Ph.D. thesis, University Pierre et Marie Curie, 169 pp .
Trenberth , K. E. , and Coauthors , 2007 : Observations: Surface and atmospheric climate change. Climate Change 2007: The Physical Science Basis, S. Solomon et al., Eds., Cambridge University Press, 235–336 .
Trzaska , S. , A. W. Robertson , J. Farrara , and C. R. Mechoso , 2007 : South Atlantic variability arising from air–sea coupling: Local mechanisms and tropical–subtropical interactions . J. Climate , 20 , 3345 – 3365 , doi: 10.1175/JCLI4114.1 .
van den Hurk , B. J. J. M. , and E. van Meijgaard , 2010 : Diagnosing land–atmosphere interaction from a regional climate model simulation over West Africa . J. Hydrometeor. , 11 , 467 – 481 , doi: 10.1175/2009JHM1173.1 .
van Oldenborgh , G. J. , F. J. Doblas-Reyes , B. Wouters , and W. Hazeleger , 2012 : Decadal prediction skill in a multi-model ensemble . Climate Dyn. , 38 , 1263 – 1280 , doi: 10.1007/s00382-012-1313-4 .
Vellinga , M. , A. Arribas , and R. Graham , 2013 : Seasonal forecasts for regional onset of the West African monsoon . Climate Dyn. , 40 , 3047 – 3070 , doi: 10.1007/s00382-012-1520-z .
Venegas , S. A. , L. A. Mysak , and D. N. Straub , 1997 : Atmosphere–ocean coupled variability in the South Atlantic . J. Climate , 10 , 2904 – 2920 , doi: 10.1175/1520-0442(1997)010<2904:AOCVIT>2.0.CO;2 .
Vera , C. , and Coauthors , 2010 : Needs assessment for climate information on decadal timescales and longer . Procedia Environ. Sci. , 1 , 275 – 286 , doi: 10.1016/j.proenv.2010.09.017 .
Vizy , E. K. , and K. H. Cook , 2001 : Mechanisms by which Gulf of Guinea and eastern North Atlantic sea surface temperature anomalies can influence African rainfall . J. Climate , 14 , 795 – 821 , doi: 10.1175/1520-0442(2001)014<0795:MBWGOG>2.0.CO;2 .
Vizy , E. K. , and K. H. Cook , 2002 : Development and application of a mesoscale climate model for the tropics: Influence of sea surface temperature anomalies on the West African monsoon . J. Geophys. Res. , 107 ( D3 ), 4023 , doi: 10.1029/2001JD000686 .
Vizy , E. K. , and K. H. Cook , 2012 : Mid-twenty-first-century changes in extreme events over northern and tropical Africa . J. Climate , 25 , 5748 – 5767 , doi: 10.1175/JCLI-D-11-00693.1 .
Vizy , E. K. , K. H. Cook , J. Crétat , and N. Neupane , 2013 : Projections of a wetter Sahel in the twenty-first century from global and regional models . J. Climate , 26, 4664–4687 , doi: 10.1175/JCLI-D-12-00533.1 .
Wagner , R. G. , and A. M. da Silva , 1994 : Surface conditions associated with anomalous rainfall in the Guinea coastal region . Int. J. Climatol. , 14 , 179 – 199 , doi: 10.1002/joc.3370140205 .
Wang , C. , 2002 : Atlantic climate variability and its associated atmospheric circulation cells . J. Climate , 15 , 1516 – 1536 , doi: 10.1175/1520-0442(2002)015<1516:ACVAIA>2.0.CO;2 .
Wang , C. , S. Dong , A. T. Evan , G. R. Foltz , and S. K. Lee , 2012 : Multidecadal covariability of North Atlantic sea surface temperature, African dust, Sahel rainfall, and Atlantic hurricanes . J. Climate , 25 , 5404 – 5415 , doi: 10.1175/JCLI-D-11-00413.1 .
Wang , G. L. , E. A. B. Eltahir , J. A. Foley , D. Pollard , and S. Levis , 2004 : Decadal variability of rainfall in the Sahel: Results from the coupled GENESIS-IBIS atmosphere–biosphere model . Climate Dyn. , 22 , 625 – 637 , doi: 10.1007/s00382-004-0411-3 .
Ward , M. N. , 1998 : Diagnosis and short-lead time prediction of summer rainfall in tropical North Africa at interannual and multidecadal timescales . J. Climate , 11 , 3167 – 3191 , doi: 10.1175/1520-0442(1998)011<3167:DASLTP>2.0.CO;2 .
Washington , R. , and Coauthors , 2012 : FENNEC—The Saharan Climate System. CLIVAR Exchanges, No. 60, International CLIVAR Project Office, Southampton, United Kingdom, 31–33 .
Xue , Y. , 1997 : Biosphere feedback on regional climate in tropical North Africa. Quart. J. Roy. Meteor. Soc., 123B, 1483–1515 , doi: 10.1002/qj.49712354203 .
Xue , Y. , and M. D. Fennessy , 2002 : Under what conditions does land cover change impact regional climate? Global Desertification: Do Humans Cause Deserts? J. F. Reynolds and D. M. Stafford Smith, Eds., Dahlem University Press, 59–74 .
Xue , Y. , K. N. Liou , and A. Kasahara , 1990 : Investigation of the biogeophysical feedback on the African climate using a two-dimensional model . J. Climate , 3 , 337 – 352 , doi: 10.1175/1520-0442(1990)003<0337:IOBFOT>2.0.CO;2 .
Xue , Y. , and Coauthors , 2004a : The Sahelian climate. Vegetation, Water, Humans and the Climate, P. Kabat et al., Eds., Springer-Verlag, 59–77 .
Xue , Y. , H.-M. H. Juang , W. Li , S. Prince , R. DeFries , Y. Jiao , and R. Vasic , 2004b : Role of land surface processes in monsoon development: East Asia and West Africa . J. Geophys. Res. , 109 , D03105 , doi: 10.1029/2003JD003556 .
Xue , Y. , F. De Sales , R. Vasic , C. R. Mechoso , S. D. Prince , and A. Arakawa , 2010a : Global and seasonal assessment of interactions between climate and vegetation biophysical processes: A GCM study with different land–vegetation representations . J. Climate , 23 , 1411 – 1433 , doi: 10.1175/2009JCLI3054.1 .
Xue , Y. , and Coauthors , 2010b : Intercomparison and analyses of the climatology of the West African monsoon in the West African Monsoon Modeling and Evaluation project (WAMME) first model intercomparison experiment . Climate Dyn. , 35 , 3 – 27 , doi: 10.1007/s00382-010-0778-2 .
Yoshioka , M. , N. M. Mahowald , A. J. Conley , W. D. Collins , D. W. Fillmore , C. S. Zender , and D. B. Coleman , 2007 : Impact of desert dust radiative forcing on Sahel precipitation: Relative importance of dust compared to sea surface temperature variations, vegetation changes, and greenhouse gas warming . J. Climate , 20 , 1445 – 1467 , doi: 10.1175/JCLI4056.1 .
Zebiak , S. E. , 1993 : Air–sea interaction in the equatorial Atlantic region . J. Climate , 6 , 1567 – 1586 , doi: 10.1175/1520-0442(1993)006<1567:AIITEA>2.0.CO;2 .
Zeng , N. , J. D. Neelin , K.-M. Lau , and C. J. Tucker , 1999 : Enhancement of interdecadal climate variability in the Sahel by vegetation interaction . Science , 286 , 1537 – 1540 , doi: 10.1126/science.286.5444.1537 .
Zhang , R. , and T. L. Delworth , 2006 : Impact of Atlantic multidecadal oscillations on India/Sahel rainfall and Atlantic hurricanes . Geophys. Res. Lett. , 33 , L17712 , doi: 10.1029/2006GL026267 .
Zhang , R. , and Coauthors , 2013 : Have aerosols caused the observed Atlantic multidecadal variability? J. Atmos. Sci. , 70 , 1135 – 1144 , doi: 10.1175/JAS-D-12-0331.1 .
Zhang , Y. , J. M. Wallace , and D. S. Battisti , 1997 : ENSO-like interdecadal variability: 1900–93 . J. Climate , 10 , 1004 – 1020 , doi: 10.1175/1520-0442(1997)010<1004:ELIV>2.0.CO;2 .
Mean precipitation climatology (mm day −1 ) for 1998–2012 from TRMM 3B42 V6 product ( Huffman et al. 2007 ): (a) daily values and (b) with a 10-day smoothing applied. Values are averaged from 12°W to 6°E to avoid the precipitation maximum over the Cameroon highlands, where seasonal variations are different from those in regions to the west.
Evolution of the number of papers published in relation to “Sahelian rainfall” from the 1950s (5 papers in the 1950s and 154 in the 1960s). In the last 15 years, the papers sum 63 840. From 1950 to 1997 data are plotted as averages over each decade; from 1998 onward data are yearly plotted. Source: Google scholar ( http://scholar.google.com ). Before 1990 units are in number of papers per decade, and after 1990 the units are number of papers per year. The dates of some of the most remarkable international projects studying the Sahelian climate variability are marked in the figure.
(top) Ensemble of AGCM response to Atlantic Niño SST for four AMMA AGCMs in JAS with respect to the control run: (a) precipitation (mm day −1 ) and (b) sea level pressure (SLP; hPa) and surface winds (m s −1 ). Figure from results computed in Losada et al. (2010) . (bottom) Leading mode of (c) tropical Atlantic SST from February–May (FMAM) to September–December (SOND) and (d) summer June–September (JJAS) precipitation over West Africa during the period 1979–2003. Only summer (left) SST and (right) precipitation (std dev in mm) patterns have been shown. Red colors are positive (negative) for the SST (precipitation) fields. Figure modified from Polo et al. (2008) .
JAS warm minus cold Mediterranean Sea composites: (a) GPCC precipitation (mm) and (b) ERA-Interim specific humidity (contours; g kg −1 ) and moisture transport at 950 hPa (arrows; reference arrow is 10 m s −1 g kg −1 ). Values in colored regions and black arrows indicate 90% significance regarding a Student’s t test. Warm and cold events are identified on the basis of a standardized Mediterranean SST index (30°–40°N, 5°W–35°E) during the period 1991–2010. Warm and cold SST years correspond to 1994, 1999, and 2003 and 1993, 1996, 1997, and 2007 during which the index is >1 and <−1, respectively. The 1991–2010 period is chosen in order to exclude the turning of the Sahel precipitation trend in the 1980s and all the data are detrended.
(a),(b) Composite of anomalous observed rainfall (mm day −1 ) in years 1983, 1987, and 1997 (“warm” Pacific years) minus 1985, 1988, and 1999 (“cold” Pacific years); (c),(d) linear component of the anomalous rainfall (mm day −1 ) simulated by the four models’ ensemble mean obtained in the sensitivity experiments; (e),(f) anomalous observed sea surface temperature (K) used in the sensitivity experiments, obtained as a composite of warm Pacific years minus cold ones; (g),(h) as in (c),(d), but for the velocity potential (10 6 m 2 s −1 ) at 200 hPa and divergent wind (m s −1 ); (i),(j) as in (c),(d), but for the streamfunction (10 6 m 2 s −1 ) at 200 hPa and rotational wind (m s −1 ), all showing the average for (left) May and June and (right) July and August. Gray contours mark where the simulated anomalies are larger in magnitude than the intermodel standard deviation. Warm (cold) Pacific years were chosen as those in which the SST expansion coefficient for the first mode of covariability between Indo-Pacific SSTs and West African rainfall was above (below) the threshold of +1 (−1) std dev of the index. Figure modified from Mohino et al. (2011c) .
A 20-yr sliding window running correlation between the Niño-3 and Sahelian rainfall indices (middle grey line), the Atl3 and Sahelian rainfall indices (bottom black line), and the Atl3 and Guinea rainfall indices (top continuous line) during June–September. Modified from Losada et al. (2012) . Atl3 is defined as the SST area averaged over the region 3°N–3°S, 20°W–0°. Niño-3 is defined as the SST area averaged over the region 5°N–5°S, 150°–90°W. The Guinea index is defined as the rainfall area averaged over the region 8°–4°N, 20°W–10°E and the Sahelian index is defined as the rainfall area averaged over the region 20°–10°N, 20°W–10°E. Dots indicate the 20-yr windows in which the correlation is significant at 95% of confidence level.
Seasonal (July–September) anomalies of rainfall over the Sahel (10°–20°N, 10°W–10°E) calculated from the CRU time series, version 3.1 (TS3.1), dataset ( Harris et al. 2014 ) (black solid line), an average of 16 AGCM models forced with observed SSTs (dark gray solid line), CanAM4 (light gray solid line), and IPSL-CM5A-MR (light gray dotted line). Data have been smoothed with a 5-yr running mean to focus on decadal time scales. Dashed dark gray lines show the one standard deviation of the 16 CMIP5 AMIP models. Simulated data were obtained from the CMIP5 database. AMIP simulations in CMIP5 start in 1979. Only two models started in 1950s, which are plotted separately in the figure.
Land surface: reductions of simulation error (mm day −1 ) by introducing the VBP over different regions in different seasons [modified from Xue et al. (2010a) ].
Average time–latitude propagation of zonally averaged (10°W–10°E) rainfall over the West African monsoon region for observations and for six state-of-the-art coupled models from ENSEMBLES. The onset date for each model is shown as a vertical black line. For reference, the average onset date in observations is 29 June. [From Vellinga et al. (2013) ]
Decadal prediction: anomaly correlation coefficient (ACC) between the Sahelian precipitation index (10°–20°N, 15°W–15°E) from the CRU dataset and decadal hindcasts (solid), historical experiments (dashed), and decadal-forcing residuals (dotted). ACC is computed for the multimodel ensemble mean. Dots indicate the 95% significant positive correlations; significance is estimated using a Monte Carlo test with 200 permutations. The decadal-forcing residuals are analyzed to obtain an evaluation of the initialization weight in the decadal experiments [see Gaetani and Mohino (2013) for details].
Future projections: summer (JAS) rainfall changes (mm day −1 ) as simulated by the (a) CMIP3 and (b) CMIP5 multimodel ensemble means. Changes are calculated between the SRES A1B scenario and historical experiments for CMIP3 and the RCP4.5 scenario and the historical experiments for CMIP5 (2065–95 vs 1970–99). The stippling indicates grid boxes where 50% of the models simulate either positive or negative changes. (c) JAS Sahel rainfall indices for the CMIP3 and CMIP5 multimodel ensembles and the CRU TS3.1 observations (see the box for the domain). The time series are in units of mean JAS rainfall where the mean is calculated for the period 1901–99. The one standard deviation spread is shown by the orange envelope for the CMIP5 ensemble and by the blue dotted lines for the CMIP3 ensemble. All indices have been low-pass filtered using an 8-yr running average [modified from Biasutti (2013) ].
Projection of the anomalous SSTs onto the grey bottom correlation line in Fig. 6 . Only 95% significant regions under a Monte Carlo test are represented.
- View raw image
- Download Powerpoint Slide
Related Content
The impacts of interannual climate variability on the declining trend in terrestrial water storage over the tigris–euphrates river basin, evaluation of snowfall retrieval performance of gpm constellation radiometers relative to spaceborne radars, a 440-year reconstruction of heavy precipitation in california from blue oak tree rings, quantifying the role of internal climate variability and its translation from climate variables to hydropower production at basin scale in india, extreme convective rainfall and flooding from winter season extratropical cyclones in the mid-atlantic region of the united states.
- Previous Article
- Next Article
Variability and Predictability of West African Droughts: A Review on the Role of Sea Surface Temperature Anomalies
Displayed acceptance dates for articles published prior to 2023 are approximate to within a week. If needed, exact acceptance dates can be obtained by emailing [email protected] .
- Download PDF
The Sahel experienced a severe drought during the 1970s and 1980s after wet periods in the 1950s and 1960s. Although rainfall partially recovered since the 1990s, the drought had devastating impacts on society. Most studies agree that this dry period resulted primarily from remote effects of sea surface temperature (SST) anomalies amplified by local land surface–atmosphere interactions. This paper reviews advances made during the last decade to better understand the impact of global SST variability on West African rainfall at interannual to decadal time scales. At interannual time scales, a warming of the equatorial Atlantic and Pacific/Indian Oceans results in rainfall reduction over the Sahel, and positive SST anomalies over the Mediterranean Sea tend to be associated with increased rainfall. At decadal time scales, warming over the tropics leads to drought over the Sahel, whereas warming over the North Atlantic promotes increased rainfall. Prediction systems have evolved from seasonal to decadal forecasting. The agreement among future projections has improved from CMIP3 to CMIP5, with a general tendency for slightly wetter conditions over the central part of the Sahel, drier conditions over the western part, and a delay in the monsoon onset. The role of the Indian Ocean, the stationarity of teleconnections, the determination of the leader ocean basin in driving decadal variability, the anthropogenic role, the reduction of the model rainfall spread, and the improvement of some model components are among the most important remaining questions that continue to be the focus of current international projects.
West Africa is the westernmost region of the northern tropical African continent. The region is primarily characterized by distributions of rainfall and vegetation that are primarily zonal with strong north–south gradients, and it is considered as an entity in the meteorological context ( Nicholson 2013 ). A monsoon season [the West African monsoon (WAM)] occurs every year, lasting from four to five months (May–September) near the Guinean coast and three months [July–September (JAS)] in the Sahel. Enhanced precipitation is associated with the seasonal northward migration of the intertropical convergence zone (ITCZ), where the northeasterly harmattan winds converge with the moisture-laden flow from the colder eastern equatorial Atlantic Ocean. Nicholson (2013) recently conducted an extensive review of rainfall variability over the Sahel and documented novel features of the region’s storm circulations. Figure 1 (from Huffman et al. 2007 ) shows a latitude–time plot that illustrates the seasonal cycle of rainfall in West Africa. Rainfall rates increase along the Guinean coast of Africa (approximately 4°–6°N) throughout May, and precipitation remains high in this region through June. In the early summer [7 July, according to climatology compiled with the Tropical Rainfall Measuring Mission (TRMM)], rainfall decreases along the coast of the Gulf of Guinea and the rainfall maximum becomes established over the Sahel (about 10°–15°N). This sudden jump in latitude of the precipitation maximum represents the onset of the West African monsoon ( Le Barbe et al. 2002 ; Sultan and Janicot 2003 ).

Citation: Journal of Climate 28, 10; 10.1175/JCLI-D-14-00130.1
- Download Figure
- Download figure as PowerPoint slide
The number of scientific papers motivated by different aspects of Sahel rainfall has increased exponentially since the 1950s (see Fig. 2 ), from around 150 to more than 5000 entries in the period from January to May 2013. Droughts are major natural disasters for the largely rain-fed agriculture of most African countries. Particularly in the Sahel, a weak rainy season can create dramatic situations for millions of people (according to the International Federation of Red Cross and Red Crescent Societies; http://www.ifrc.org ). The Sahel drought during the 1970s and 1980s was the most significant climate event at the continental scale during the twentieth century, and is arguably among the largest climatic changes worldwide ( Trenberth et al. 2007 ). The event was associated with changes in the intensity, spatial distribution, and temporal evolution of the WAM and associated circulation features, such as the trade winds, African easterly jet (AEJ), and tropical easterly jet (TEJ) ( Le Barbe et al. 2002 ; Sultan and Janicot 2003 ; Xue et al. 2004a ; Dezfuli and Nicholson 2011 ).

The rainy season in the Sahel has large interannual and decadal variations. A substantial part of this variability is due to the influence of slowly varying climate subcomponents, such as sea surface temperatures (SSTs) and land surface conditions. The importance of oceanic influences at interannual and decadal time scales has been supported by the results of several studies ( Folland et al. 1986 ; Palmer 1986 ; Rowell et al. 1992 ; Ward 1998 ; Camberlin et al. 2001 ; Giannini et al. 2003 ; Lu and Delworth 2005 ; Cook 2008 ; Caminade and Terray 2010 ; Losada et al. 2010 ; Rodríguez-Fonseca et al. 2011 ; Mohino et al. 2011a ; Rowell 2013 ; Nicholson 2013 ). Other studies have addressed the effects of land–atmosphere interactions ( Xue, 1997 ; Zeng et al. 1999 ; Nicholson 2000 ; Giannini et al. 2003 ; Yoshioka et al. 2007 ) and aerosol–radiative forcings ( Kim et al. 2010 ). These effects can potentially interact with each other. For example, the variability of land surface conditions can affect the circulation over the ocean, which in turn can modify the SSTs and indirectly affect conditions over land ( Ma et al. 2013 ).
The existence of significant impacts on WAM rainfall of slowly varying climate subcomponents indicates the potential for useful long-range forecasts ( Vellinga et al. 2013 ; Gaetani and Mohino 2013 ; García-Serrano et al. 2013 ). To realize this potential with climate models, these must successfully reproduce the important characteristics of the WAM precipitation and circulation. Despite continuous model improvements in the models, a skillful simulation and prediction of the WAM, including its variability at different time and spatial scales and its association with external forcings, remains a daunting task.
The present paper surveys the literature on drought in West Africa and the Sahel with particular emphasis on recent work on these subjects. The text discusses the dynamical mechanisms linking anomalies in West African rainfall with those in SST over the World Ocean, the time dependence of these relationships, their predictability, and future projections. It is appropriate to acknowledge that many results presented in the following were obtained under the sponsorship of coordinated international research projects (see Fig. 2 ). The African Monsoon Multidisciplinary Analysis program (AMMA; http://amma-international.org/ ) has coordinated an ambitious program aimed to improve the knowledge and understanding of the WAM’s variability and predictability on daily-to-decadal time scales, including climate change ( Redelsperger et al. 2006 ; AMMA 2010 ; Ruti et al. 2011 ). The West African Monsoon Modeling and Evaluation (WAMME; Druyan et al. 2010 ) is a community initiative designed to evaluate the performance of state-of-the-art GCMs and regional climate models (RCMs) in reproducing WAM precipitation, drought scenarios, and their relevant processes. WAMME applies recently available observational and assimilation data for model evaluation and improvement ( Boone et al. 2010 ; Xue et al. 2010b ). The Coordinated Regional Climate Downscaling Experiment in Africa (CORDEX-Africa) has led to a coordinated evaluation of RCM skill, spread and uncertainties for simulating the West African monsoon mean climate and, to a lesser extent, its simulated onset and variability ( Nikulin et al. 2012 ; Hernández-Díaz et al. 2013 ). The European Commission Seventh Framework Programme (EC FP7) Quantifying Weather and Climate Impacts on Health in Developing Countries (QWECI) project has aimed to understand, on a more fundamental level, the climate drivers of the vector-borne diseases of malaria, Rift Valley fever, and certain tick-borne diseases, all of which have major human and livestock health and economic implications in Africa ( Cash et al. 2013 ; Tompkins and Ermert 2013 ; Ermert et al. 2013 ; Caminade et al. 2014 ). Some of these international projects have focused on the impact of SST anomalies on the WAM at interannual and decadal time scales. The collective findings from research sponsored by these projects have contributed significantly to progress, particularly in cases that occurred in the last few decades. Finally, phases 3 and 5 of the Coupled Model Intercomparison Project (CMIP3 and CMIP5) have addressed outstanding scientific questions in the Intergovernmental Panel on Climate Change (IPCC) Fourth and Fifth Assessment Reports (AR4 and AR5) process, improving understanding of climate and providing estimates of future climate change that will be useful to those considering its possible consequences.
The text is organized as follows. We start in section 2 and 3 by surveying the state-of-the-art knowledge of the SST influence on Sahel rainfall at interannual to decadal time scales, at which the variability of the ocean is the main driver of that in the atmosphere. Next, we summarize the progress in seasonal ( section 5 ) to decadal predictability ( section 6 ) and its skill in West Africa, following with an update of the future projections. A final section will summarize the most remarkable results, remaining questions, modeling issues, and future directions (section 8).
2. SST influence at interannual time scales
This section is dedicated to review recent findings on the influence of SST anomalies in different ocean basins (the Atlantic, Pacific, and Indian Oceans and the Mediterranean Sea) on the WAM precipitation at interannual time scales.
Since the early papers by Hastenrath and Lamb (1977) , Lamb (1978) , and Hastenrath (1984) , many others have documented the tropical Atlantic influence on West African rainfall. This influence unfolds at different time scales: the variability in the equatorial and southern sectors affects that in interannual time scales, while that in the northern sector affects that in decadal time scales ( Hastenrath and Polzin 2011 ).
At interannual time scales, the leading mode of tropical Atlantic variability is the Atlantic Niño, also known as the equatorial mode or zonal mode ( Zebiak 1993 ; Carton et al. 1996 ). This coupled atmosphere–ocean mode is characterized by a warming (cooling) of the equatorial Atlantic during the boreal spring–summer in association with a relaxation (strengthening) of the trades and a deepening (shallowing) of the eastern equatorial thermocline. Several works using different methodologies have concluded that events of positive SST anomalies (Atlantic Niños) originate a dipole pattern of precipitation anomalies consisting of positive values along the coast of Guinea and negative ones over the Sahel ( Horel et al. 1986 ; Wagner and da Silva 1994 ; Fontaine and Janicot 1996 ; Ward 1998 ). For a warming in the Atlantic, most AGCMs show how this dipole is the result of a weakening in the sea level pressure gradient between ocean and land and hence a weaker ITCZ shift, which is translated into more rainfall over Guinea and less rainfall over Sahel ( Fig. 3 , top). In the last decades, major international field programs, such as PIRATA ( Servain et al. 1998 ), have sampled the tropical Atlantic and gathered important in situ data. Moreover, unprecedented information is been provided by instruments on board satellites. The resulting availability of homogeneous data in time and space has allowed for a better analyses of covariability between SSTs in the tropical Atlantic and rainfall in West Africa ( Ruiz-Barradas et al. 2000 ; Vizy and Cook 2002 ; Giannini et al. 2003 ; Gu and Adler 2006 ; Reason and Rouault 2006 ; Polo et al. 2008 ). On the basis of data from the end of the 1970s, Polo et al. (2008) demonstrated that an Atlantic Niño can be associated with positive rainfall anomalies over the coast and negligible ones over the Sahel, as it could be expected from the dipolar structure in precipitation anomalies reported in previous studies ( Fig. 3 , bottom). Polo et al. (2008) also found that SST anomalies along the equator in the northern summer were preceded in spring by anomalies along the Benguela coast of Angola in association with the Atlantic Niño, putting forward the potential predictability of the West African rainfall linked to the variability in the southern tropical Atlantic. Also, in this work, the presence of anomalies with different signs in the Pacific and Indian Ocean raised a hitherto unexplored possibility: the influence of these tropical oceans could interfere with the direct influence of the tropical Atlantic ( Mohino et al. 2011b ; Losada et al. 2012 ; Rodríguez-Fonseca et al. 2009 , 2011 ).

Most current state-of-the-art atmospheric GCMs (AGCMs) are able to capture the links between SST anomalies and anomalous precipitation over West Africa using either observed global SSTs ( Mohino et al. 2011b ) or observed SSTs over the tropical Atlantic with climatology elsewhere ( Vizy and Cook 2001 ; Wang 2002 ; Losada et al. 2010 ). Nevertheless, most coupled atmosphere–ocean GCMs of the current generation have important systematic errors in the tropical Pacific and Atlantic Oceans as they obtain too weak trades, a spurious ITCZ south of the equator, and too warm SSTs in the eastern part of the basins ( Mechoso et al. 1995 ; Davey et al. 2001 ; Richter et al. 2014 ; Biasutti et al. 2006 ). Such errors compromise the successful representation of the WAM and its response to the equatorial mode ( Joly and Voldoire 2010 ; Rowell 2013 ).
Complementary to works examining the impacts of SST anomalies in the tropical Atlantic, Nnamchi and Li (2011) highlighted the importance of anomalies in the subtropical South Atlantic together with temperature anomalies with the opposite sign at the equator forming a South Atlantic Ocean dipole. This configuration of SST anomalies would impact rainfall over the Gulf of Guinea coast via the so-called Lindzen–Nigam process (i.e., by enhancing anomalous divergence with opposite sign at both centers of the dipole). Venegas et al. (1997) and Trzaska et al. (2007) also discussed the relative importance of the South Atlantic in the tropical Atlantic variability.
The finding of relationships between Mediterranean climate variability and WAM dynamics motivated a new line of research in the last decade. Rowell (2003) showed that positive SST anomalies in the Mediterranean Sea tend to be associated with similarly positive precipitation anomalies in the Sahel ( Fig. 4a ). He demonstrated that increased evaporation over the positive SST anomalies leads to increased moisture content in the lower troposphere, which is advected southward into the Sahel by the low-level flow across the eastern Sahara, resulting in stronger moisture convergence and precipitation over the Sahel. Figure 4b illustrates these features using recent observed and reanalysis data. By means of AGCM sensitivity experiments, Rowell (2003) showed that such increase in rainfall is then amplified by positive feedback mechanisms, such as 1) a more intense moisture inflow from the tropical Atlantic triggered by enhanced convective heating, 2) a reduced outflow of moisture from the midlevel African easterly jet, 3) an enhanced hydrological cycle, and 4) a larger rainfall contribution by African easterly waves.

More recently, a number of empirical and numerical studies have provided further support to the links between anomalies in Mediterranean SSTs and WAM precipitation ( Jung et al. 2006 ; Fontaine et al. 2011b ; Polo et al. 2011 ). Peyrillé et al. (2007) and Peyrillé and Lafore (2007) described the local circulations and mechanisms favoring the northward migration of the monsoon rainbelt. Fontaine et al. (2010) provided evidence that warm events over the Mediterranean Sea are associated with an intensified WAM, stronger low-level moisture advection, and a more northward location of ascending motions in West Africa. Moreover, they found that SST variations in the western Mediterranean Sea are associated with others in deep convection over the Gulf of Guinea, while those in the eastern Mediterranean Sea affect the atmospheric circulation over the North African subcontinent. Thus, anomalous eastern Mediterranean Sea warm conditions are linked to a northward migration of the monsoon system accompanied by enhanced southwesterly flow and weakened northeasterly climatological wind. Gaetani et al. (2010) explored these relationships at subseasonal time scales, showing that rainfall anomalies in the Sahel are concentrated in July–August when the monsoon circulation is fully developed inland, so that the effect of the northerly moisture transport from the Mediterranean Sea is maximized during that period.
At interannual time scales, a warming in the tropical Pacific tends to be associated with increased precipitation over the Gulf of Guinea and decreased precipitation over the Sahel ( Folland et al. 1986 ; Rowell et al. 1995 ; Janicot et al. 1996 , 1998 , 2001 ; Ward 1998 ; Camberlin et al. 2001 ; Rowell 2001 ; Joly et al. 2007 ). Not all analyses before the 2000s, however, were supportive of this negative link ( Kiladis and Diaz 1989 ; Ropelewski and Halpert 1989 ; Shinoda and Kawamura 1994 ).
In the framework of AMMA, experiments on the sensitivity of WAM rainfall to SST anomalies in the Pacific were performed for the period 1979–2002. In general, the results showed that positive SST anomalies in the Pacific SST have negative effects on Sahel rainfall ( Mohino et al. 2011c ). Figure 5 shows composites of rainfall differences between years with both warmer and colder than average SSTs in the Pacific Ocean ( Mohino et al. 2011c ). The relationships between SST anomalies in the Pacific and Indian Oceans with West African rainfall vary strongly with season, being different for May–June ( Fig. 5 , left) and for July–August ( Fig. 5 , right). In late spring, anomalous subsidence develops over both the Maritime Continent and the equatorial Atlantic in association with enhanced equatorial heating in the Pacific. Rowell (2001) interprets this feature as a stationary Kelvin wave response originating in the east Pacific. Precipitation increases over continental West Africa in association with stronger zonal convergence of moisture. In addition, precipitation decreases over the Gulf of Guinea. During the monsoon peak (July and August), the SST anomalies move westward over the equatorial Pacific and the two regions of subsidence during the previous months merge over West Africa, weakening the monsoon and thus rainfall over the Sahel.

It has been shown using observational data that links between anomalies in WAM rainfall and Pacific SSTs occur during the developing phase of an El Niño–Southern Oscillation (ENSO) event. That is, the anomaly in WAM appears in boreal summer before the peak of ENSO in autumn–winter. CGCMs have difficulties in capturing the temporal aspects of these connections, as shown by Joly and Voldoire (2009) for CMIP3 models. These model difficulties were attributed to shortcomings in the simulation of ENSO locking to the seasonal cycle and the associated atmospheric teleconnections.
The Indian Ocean has important impacts on the Sahel at both decadal and interannual time scales. At interannual time scales, Biasutti et al. (2008) found substantial negative correlations between Sahel precipitation and Indian Ocean SSTs in observations and in CGCM simulations performed in the framework of CMIP3. Bader and Latif (2011) argued that the Indian Ocean SSTs were the main forcing for the drought over the western Sahel in 1983, presenting evidence that the dry conditions that persisted over the western Sahel in that year were mainly forced by positive SST anomalies in the Indian Ocean, which probably remained from the strong 1982/83 El Niño event. Except for 1983, however, SST anomalies in the Indian Ocean at interannual time scales are generally weak in comparison to the other basins. Thus, the impact of Indian Ocean anomalies on Sahel rainfall could be masked by that of other basins. Palmer (1986) analyzed the AGCM response to SST anomalies in individual ocean basins that correspond to those in the SST pattern for the global tropics used in Folland et al. (1986) . The AGCM experiments showed that the Indian Ocean warming produces a slight rainfall enhancement over the western Sahel, but that the concomitant anomalies in the Atlantic and Pacific Oceans are responsible for the precipitation reduction in the west Sahel. Fontaine et al. (2011a) showed that positive SST differences between the Indian Ocean and the eastern Mediterranean Sea are synchronous with in-phase rainfall deficits over the whole Sudan Sahel. In addition, Rowell (2001) suggested that the impact of the Indian Ocean acts through changes in the large-scale gradient in SSTs from the west Pacific to the Indian Ocean. AGCM experiments showed that these changes lead to a stationary equatorial Rossby wave response over the Indian Ocean, causing anomalous subsidence over the Sahel.
Studies performed in the last decades have argued that the impact of SST anomalies in some basins appear to be different depending on the decades considered. We will refer to this property as “nonstationarity.”
The links between SST anomalies in the equatorial Atlantic and rainfall over the Gulf of Guinea are persistent features, but those with the Sahel rainfall seem to wane after the 1970s and at the beginning of the twentieth century. In these periods, the observations suggest that the influence of the Atlantic SST anomalies on the Sahel rainfall is balanced by concomitant SST anomalies with opposite signs in the Pacific and Indian Oceans (see Fig. 6 ). Joly and Voldoire (2010) , Rodríguez-Fonseca et al. (2011) , and Mohino et al. (2011b) have suggested that the counteracting effect of the Pacific could explain the absence of the dipolar relation with the Guinean rainfall reported before the 1970s. This hypothesis has been recently validated by the sensitivity experiments performed by Losada et al. (2012) with different AGCMs with prescribed SSTs in single or multiple ocean basins.

A similar tendency to nonstationarity appears in the impact of SST anomalies in the Mediterranean Sea. After removing the global SST trend during the twentieth century, Fontaine et al. (2011a) indicated that SST anomalies in the eastern Mediterranean Sea and Indian Ocean have important effects on WAM rainfall, both in terms of intensity and time stability, with a growing importance of the Mediterranean Sea at the end of the twentieth century.
Broadly, two explanations have been offered for the nonstationarity of impacts. First, nonstationarity might be simply an artifact of sampling errors because some variation in the correlation between two variables can be expected by chance even during a stationary period of climate. Rowell (2013) addressed this hypothesis by applying a bootstrap resampling technique to SST teleconnections to African rainfall. He found no reason to reject the hypothesis that nonstationarity and multidecadal variability in the strength of these teleconnections might arise only from sampling variability, at least for the specific SST and rainfall in the regions considered. Internal atmospheric variability could also be relevant even at these longer time scales. For instance, Traore (2011) showed that running a AGCM over one to several centuries with fixed SST patterns (either the 1955–65 “moist” SST pattern or 1975–85 “dry” pattern) can provide decadal-scale rainfall anomalies up to 20% of the difference between the “moist” and “wet” long-term rainfall averages. Second, nonstationary teleconnections could be due to the slowly varying oceans and their multidecadal modes, as will be discussed later. These can alter the mean state of the atmosphere and/or the magnitude of SST variability in a way that during some decades the Atlantic influence interferes with that from the Pacific, whereas in other decades the Atlantic influence acts in isolation from the rest of the tropical basins ( Mohino et al. 2011b ).
3. Variability at decadal time scales
The first hypothesis on the prolonged Sahelian drought was that a decrease in vegetation cover led to increased albedo and, consequently, to enhanced subsidence in the region ( Charney 1975 ). Later studies, however, identified the crucial role of SSTs over the world ocean in driving decadal Sahelian rainfall variability at decadal time scales. Using observed SSTs as boundary conditions, most AGCMs are able to reproduce the twentieth-century drying trend in West Africa and the Sahel drought of the 1970–80s and subsequent rainfall recovery ( Rowell 1996 ; Giannini et al. 2003 ; Bader and Latif 2003 ; Lu and Delworth 2005 ; Haarsma et al. 2005 ; Hoerling et al. 2006 , 2010 ; Lu 2009 ; Tippett 2006 ; Tippett and Giannini 2006 ; Caminade and Terray 2010 ; Mohino et al. 2011a ; see also Fig. 8 ). The AGCM simulated impacts of SST anomalies on Sahel rainfall at decadal time scales, however, are weaker in comparison to the observations. This might be partially as a result of many reasons, such as shortcomings in the representation of vegetation–land–atmosphere interactions ( Zeng et al. 1999 ; Giannini et al. 2003 ; Wang et al. 2004 ; Kucharski et al. 2013 ), changes in surface albedo ( Kucharski et al. 2013 ), feedback with desert dust ( Yoshioka et al. 2007 ; Wang et al. 2012 ; Mahajan et al. 2012 ; Chin et al. 2014 ), and the atmosphere’s internal variability ( Traore 2011 ). Analyses carried out by the International Climate of the Twentieth Century Project (C20C) confirmed that SST variability is one, but not the only, driver of past Sahel droughts ( Scaife et al. 2009 ). In this section we discuss separately the role of SST anomalies and land surface processes on the Sahel drought.
Decreased Sahel rainfall at decadal time scales has been associated with the warming of the tropical SSTs ( Giannini et al. 2003 , 2013 ; Lu and Delworth 2005 ; Mohino et al. 2011a ). Hagos and Cook (2008) suggested that the combination of the SST anomalies over the tropical Indian and Atlantic Ocean basins was responsible for the 1980s Sahel drought and subsequent recovery in the 1990s. Caminade and Terray (2010) highlighted the role of the warming of the Pacific basin in reducing Sahel rainfall, while other works suggested that the drought was driven by the warming trend of the Indian Ocean basin during the late twentieth century ( Bader and Latif 2003 ; Giannini et al. 2003 ; Tippett and Giannini 2006 ; Lu 2009 ). This warming of the tropical Indian Ocean and/or Pacific basin would induce enhanced convection locally and the propagation of Kelvin and Rossby waves that would communicate the tropospheric warming to West Africa ( Chiang and Sobel 2002 ; Lu 2009 ). This would stabilize the region, leading to decreased rainfall over the Sahel through the so-called upped-ante mechanism ( Neelin et al. 2003 ; Lu 2009 ; Caminade and Terray 2010 ). Lu and Delworth (2005) also suggested that the Pacific and Indian Ocean warming could lead to an anomalous Walker-type overturning cell with increased dry air subsidence over the Sahel and subsequent drought.
Alternatively, Sahel drought at decadal time scales has been related to the impacts of an interhemispheric dipole pattern of SST anomalies that is global, but most pronounced in the Atlantic basin ( Folland et al. 1986 ; Palmer 1986 ). The differential heating of the Northern and Southern Hemisphere leads to a meridional shift of the ITCZ ( Zhang and Delworth 2006 ; Kang et al. 2009 ; Hwang et al. 2013 ) and anomalous rainfall in the Sahel ( Hoerling et al. 2006 ; Knight et al. 2006 ; Ting et al. 2009 , 2011 ; Mohino et al. 2011a ). Mechanistically, the movement of the rainbelt in the Sahel might occur through changes in the moisture content of the monsoon flow ( Giannini et al. 2013 ) or circulation changes linked to a strengthening of the Saharan heat low ( Martin and Thorncroft 2014a ).
The relative importance of SST anomalies in different basins on Sahel rainfall has been greatly debated (e.g., Hoerling et al. 2006 ; Giannini et al. 2003 ; Bader and Latif 2003 ), partly because the sensitivity to each basin appears to be highly model dependent ( Scaife et al. 2009 ; Biasutti et al. 2008 ) and partly because the details of the warming pattern matter greatly ( Hagos and Cook 2008 ). Yet, recent work by Giannini et al. (2013) suggests that these differences might be reconciled by assuming that Sahel rainfall responds to relative changes between SST anomalies in the northern Atlantic and the entire tropics.
The broad agreement of the scientific community on SST anomalies being crucial drivers of Sahel rainfall decadal variability during the twentieth century has spurred a lively debate on whether this variability might be due to external forcings (anthropogenic and natural emissions) or to internal climate processes (natural variability modes) or, more likely, to a combination of the two. Recently, Mohino et al. (2011a) interpreted the pattern of SST variations linked to SST rainfall changes as a combination of three different modes of SST decadal variability: the response to the global warming trend, the positive phase of the interdecadal Pacific oscillation ( Zhang et al. 1997 ), also known as the Pacific decadal oscillation ( Mantua et al. 1997 ), and the negative phase of the Atlantic multidecadal oscillation (AMO; Knight et al. 2005 ), with all three leading to reductions in Sahel precipitation. Some works suggests that the observed AMO is an internal mode of variability not explained by external forcings ( Knight et al. 2005 ; Knight 2009 ; Ting et al. 2009 ; 2011 ), while others point to the role of anthropogenic aerosols in cooling the North Atlantic more than the South Atlantic ( Rotstayn and Lohmann 2002 ; Biasutti and Giannini 2006 ; Kawase et al. 2010 ; Ackerley et al. 2011 ; Biasutti 2011 ). Recently, Booth et al. (2012) showed that their CGCM experiments could simulate most of the observed twentieth-century SST variability in the North Atlantic, and that this variability was highly dependent on the indirect effect of aerosols. However, Zhang et al. (2013) call into question these results as there are multiple inconsistencies between the previous experiments and key aspects of observed variability within and without the North Atlantic.
Hoerling et al. (2006) and Lau et al. (2006) analyzed historical simulations of CGCMs participating in the CMIP3. Overall, the models failed to simulate the mid-twentieth-century Sahel drought and recent recovery ( Hoerling et al. 2010 ) with the correct magnitude and timing, suggesting that anthropogenic forcings played little or no role in driving the drought. On the other hand, Held et al. (2005) showed that the GFDL CM2.0 and CM2.1 coupled models could very well reproduce the observed drying trend during the second half of the twentieth century. Ackerley et al. (2011) suggested that although the aerosols contributed to the intense decline in the rainfall over the Sahel in the 1950–80 period, a fraction of this drying could be related to either the effect of an internal mode (AMO) or climate model deficiencies. Biasutti and Giannini (2006) estimated from historical CMIP3 simulations that anthropogenic forcing, especially by aerosols, may have contributed a third of the long-term twentieth-century drying, and that estimate has been confirmed by CMIP5 models ( Biasutti 2013 ). Recently, Hwang et al. (2013) posited that both the drought and subsequent recovery in the Sahel are greatly influenced by the increase and subsequent reduction of sulfate emissions in Europe and North America. However, neither generation of CMIP climate models reproduces the magnitude of the observed rainfall variability at decadal to multidecadal time scales over the twentieth century. Dismissing a strong role of natural variability in driving droughts at time scales less than a century might thus be premature ( Biasutti 2011 ). This point of view is strengthened by paleoclimate and historical studies, which have highlighted how recent droughts in the Sahel are not unprecedented ( Shanahan et al. 2009 ; Brooks 1998 ; Nicholson 1978 , 1979 ; Nicholson et al. 2012 ). Shanahan et al. (2009) highlighted the existence of intervals of severe drought lasting for periods ranging from decades to centuries over Ghana over the last 3000 years and they related these megadroughts to the AMO. Prolonged drought episodes lasting from 1100 to 1500 might have partly contributed to the collapse of the Malian empire ( Brooks 1998 ); multidecadal droughts were also reported in Senegambia from 1710 to 1750 and from 1770 to 1780 followed by reported mass starvation from 1790 to 1840 ( Nicholson 1978 , 1979 , 2013 ). The Sahel drying observed during the second half of the twentieth century appears to be neither unusual nor extreme from a paleoclimate perspective or considering the long-term historical context.
Analyses of simulations by recent generations of CGCMs have provided additional insight on the WAM and its variability ( Fig. 7 ). Control runs, historical simulations, future projections, and seasonal-to-decadal predictions have been analyzed. Rowell (2013) has recently assessed the capability of Sahel models to represent WAM teleconnections. His work suggests that some teleconnections tend to be poorly reproduced (e.g., those with the equatorial Atlantic SSTs and the Pacific ENSO), while others seem to be captured by most models (e.g., the link between the Mediterranean SSTs and Sahel rainfall).

Pioneer projects as Hydrological Atmospheric Pilot Experiment (HAPEX) in the Sahel ( Goutorbe et al. 1997 ) made a contribution to understanding the land surface processes in relation to the WAM variability. Since then, several studies have addressed the role of land surface processes in driving Sahel drought. Land surface parameterizations in the atmospheric models used in these studies cover a wide range of complexity (e.g., Laval and Picon 1986 ; Sud and Molud 1988 ; Xue et al. 1990 ; Eltahir and Gong 1996 ). Nevertheless, the results on sensitivity to land conditions in Sahel were quite consistent across models. Increases in albedo (soil moisture) produce negative (positive) feedbacks on rainfall, even the magnitude of the impacts is within a narrow range ( Xue and Fennessy 2002 ). A proper evaluation of the surface feedback to climate can be obtained only when all relevant components of the surface energy and water balances are taken into account.
The impact of land degradation upon regional climate seasonal variability and drought events over the Sahel has been explored using a GCM coupled to a biophysical model ( Xue et al. 2004a ). According to the results, the primary effect of degrading savanna and shrub conditions in the Sahel is reduced evaporation. This is partially due to reduced net radiation because of higher albedo, but more importantly to lower leaf area index (LAI) and surface roughness length, and to higher stomatal resistance. The reduction in evaporation results in less convection and lower latent heating rates in the troposphere, in association with a relative subsidence, which in turn weakens the monsoon flow and reduces moisture flux convergence and lowers rainfall.
It has also been found that the Sahel, along with a few other regions that are mostly semiarid, has the largest soil moisture/climate coupling strength in the world. This result was obtained in the Global Land–Atmosphere Coupling Experiment (GLACE; Koster et al. 2006 ). Two sets of boreal summer (June–August) simulations were performed with multiple GCMs. In one, moisture varied during the simulations, while in the other set the same geographically varying time series of subsurface soil moisture was prescribed. The soil moisture–atmosphere coupling strength in each model was then estimated with a statistical method. The high soil moisture–climate coupling strength in the Sahel is consistent with a number of soil moisture feedback studies (e.g., Douville 2002 ; Philippon and Fontaine 2002 ). Results based on CMIP3 simulations also show that North Africa is another region with strong soil moisture feedbacks ( Notaro 2008 ). A few modeling studies have shown that the root-zone soil moisture might not act as a memory of rainfall anomalies for the following rainy season and therefore might not affect the persistence of the drought ( Shinoda and Yamaguchi 2003 ; Douville, et al. 2007 ; van den Hurk and van Meijgaard 2010 ).
The impact of the vegetation biophysical processes (VBP; i.e., land surface processes relevant to climate interactions associated with vegetation) on the WAM has also been investigated by Xue et al. (2004b , 2010b) , who analyzed the results from two AGCMs coupled to three different land models with varying degrees of physically based complexity in the representation of VBP. The criterion to assess the importance of VBP effects was based on the simulation skill in reproducing the observed global precipitation under the assumption that their inclusion would improve precipitation simulations. Figure 8 shows the reduction in absolute seasonal mean bias of 5-yr mean simulated precipitation (or improved prediction skill) due to VBP processes. Accordingly, West Africa has the largest VBP impact in the world, especially in summer (see also Ma et al. 2013 ). Using remotely sensed LAI datasets, compared with using LAI based on a few ground surveys, in the boundary conditions of a GCM produced substantial improvements in the near-surface climate in West Africa ( Kang et al. 2007 ; Li et al. 2007 ).

The numerical experiments described so far in this section prescribe land conditions. Other experiments have also been performed allowing for two-way vegetation–climate interactions (i.e., land surface conditions are not specified but predicted). Using a simple dynamic vegetation model coupled with the Quasi-Equilibrium Tropical Circulation Model (QTCM), Zeng et al. (1999) showed that the best reproduction of the observed interannual precipitation variability over the Sahel during the past half century was obtained when interactions among vegetation, soil, and ocean components were all included. Wang et al. (2004) investigated the impact of large-scale oceanic forcing and local vegetation feedback on the variability of Sahel rainfall using a global biosphere–atmosphere model. When vegetation was dynamic, the model realistically reproduced the multidecadal-scale fluctuation of rainfall in the Sahel region. However, when the vegetation was kept static, the rainfall regime was characterized by fluctuations at much shorter time scales. This suggests that vegetation dynamics acts as a mechanism for the persistence of the regional climate. Kucharski et al. (2013) obtained a similar result and showed that about 60% of the observed Sahel drought could be reproduced if vegetation dynamics were included in their AGCM ensemble simulations, whereas only 30% could be reproduced if vegetation feedbacks were static. Furthermore, Kucharski et al. (2013) demonstrated that the dominant positive feedback mechanism for the vegetation impact on the Sahel drought is the albedo feedback, in accordance with early work by Charney (1975) .
Although land use changes might not have been the main driver of the 1980s Sahel drought, their impacts over West Africa could increase on the coming years ( Taylor et al. 2002 ). In fact, the work by Paeth et al. (2009) suggests that in climate change scenarios land use changes could become primarily responsible for the simulated climate response over West Africa.
During the last decade seasonal forecasting has matured from a research activity to a fully operational service, with many centers using initialized state-of-the-art coupled models. There are currently 12 WMO Global Producing Centers (GPCs) for long-range forecasting that routinely issue operational forecasts for rainy season totals ( Graham et al. 2011 ). The forecasts are freely available for national meteorological services, regional climate centers, and global product centers via www.wmolc.org .
Over the African region, the skill of these long-range forecasting systems to predict seasonal total rainfall is high enough to make them useful for planning purposes. An example of the practical use of long-range forecasts and postprocessing techniques is the Prévisions Saisonnière en Afrique de l’Ouest (PRESAO; Fig. 9 , top) forum, which meets annually to produce a consensus forecast for the West African region. To make this forecast, output from GPC dynamical systems and statistical models are combined and a spatial bias correction is made using canonical correlation analysis.

These improvements in forecasting seasonal averages have been achieved thanks to the continuous research completed in the last 10 years. For example, Philippon et al. (2010) found that the correlation between observations and a five-model ensemble mean from the ENSEMBLES Sixth Framework Programme (FP6) project ( Hewitt 2004 ) was 0.55 for the Guinean rainfall variability mode. Batté and Déqué (2011) also used the ENSEMBLES dataset to analyze the skill of the multimodel system not only for the WAM but also the South African winter rainy season and the greater Horn of Africa long and short rains. They found that the multimodel ensemble improved the spread-to-skill ratio and average skill score over the use of a single model by as much as 10% when measured as potential economic value. Philippon et al. (2010) and Ndiaye et al. (2011) found that skill can be enhanced by predicting modes of variability using model output statistics (MOS) instead of using direct model output and therefore such enhancements are used to produce forecasts.
Despite these advances, there are still some remaining scientific questions such as large biases in tropical Atlantic SSTs in coupled models. Also, from a user’s point of view, even more critical than the total seasonal rainfall is the ability to predict the temporal distribution of rain throughout the season ( Salack et al. 2014 ), which determines the optimal planting and harvesting time ( Marteau et al. 2011 ). This has created a clear demand for information such as the onset of rainy seasons in many parts of Africa ( Ingram et al. 2002 ; Graham et al. 2011 ).
Vellinga et al. (2013) analyzed the links between SST and precipitation over the WAM region using dynamical forecasting systems from the ENSEMBLES project. Confirming the results found by Salack et al. (2014) in observations, Vellinga et al. (2013) showed that the forecast skill in coupled models was related to tropical Atlantic SSTs in June. The ability of models to forecast the timing of the monsoon onset was found to be useful, with relative operating characteristics (ROC) scores of 0.6–0.8 at 3-months lead time.
A crucial point from the user perspective is that useful levels of skill are found even in some models with large mean rainfall biases over the region. This is important because, although there are still major model problems that need to be solved (such as biases in tropical Atlantic SSTs and total precipitation), the forecast systems are starting to be able to provide relevant probabilistic information to a crucial user question: Will the onset of the WAM be earlier or later than average this year? Figure 9 (from Vellinga et al. 2013 ) shows a major advance toward a meaningful answer to such a question, since only 10 years ago there was no forecasting system able to do it with a direct and relevant application to users.
The new field of decadal climate prediction aims to provide climate information on time scales from a few years to a few decades into the future, which is recognized as a key planning horizon ( Goddard et al. 2010 ; Vera et al. 2010 ; Smith et al. 2012 ). Decadal predictions are certainly of increasing scientific interest because they potentially represent a benefit to society through improvements in the development of climate services and adaptation strategies. They are recognized as a major part of the CMIP5 experimental design ( Taylor et al. 2012 ).
Decadal predictions explore the benefits of initializing climate models. The initialization tries to provide the forecast system with contemporaneous information on, for instance, the state of the upper-ocean heat content, to achieve forecast quality beyond that provided by simulations based only on externally forced signals such as the standard CMIP3 and CMIP5 climate change scenario experiments. The objectives of decadal prediction include capturing low-frequency internal variability and correcting the model response to climate change forcings and commitment ( Meehl et al. 2009 , 2014 ; Murphy et al. 2010 ; Goddard et al. 2013 ; Doblas-Reyes et al. 2013 ).
As has been indicated in previous sections, WAM variability at decadal time scales results from a combination of internal and externally forced components ( Biasutti 2011 ; Mohino et al. 2011a ), including anthropogenic aerosols (e.g., Biasutti and Giannini 2006 ) and greenhouse gases (e.g., Biasutti et al. 2008 ). All the evidence points to the WAM as a good test bed for assessing the feasibility of decadal prediction.
Using the first coordinated experiment to explore the feasibility of decadal prediction (ENSEMBLES; Doblas-Reyes et al. 2010 ), van Oldenborgh et al. (2012) and MacLeod et al. (2012) found no significant skill in point-wise precipitation predictions over West Africa for 4-yr forecast averages. Predictions of precipitation trends did not have skill either. MacLeod et al. (2012) further suggested that the spread of precipitation hindcasts over land is such that the potential for predictability may not be sufficient to be useful. However, García-Serrano et al. (2013) have shown that the ENSEMBLES forecast systems are reliable, in a probabilistic sense, when recapturing the Sahelian precipitation variability. Instead, multiyear forecast quality assessment of the dominant WAM precipitation regimes suggests that the Guinean rainfall is not skillful.
From the most recent generation of climate models (i.e., CMIP5), Goddard et al. (2013) , Gaetani and Mohino (2013) , and Doblas-Reyes et al. (2013) found positive pointwise precipitation correlation over West Africa. However, this finding is model dependent, and the conditional biases that exist in the predictions may actually make the information less accurate than climatological averages ( Goddard et al. 2013 ). A proper simulation of the remote SST influences on the Sahel appears to be key for predictability at decadal–multidecadal time scales ( Martin and Thorncroft 2014b ). The Sahelian rainfall skill is likely associated with a significant skill in the AMO prediction ( García-Serrano et al. 2015 ; Guemas et al. 2015 ), although the relative SST difference between the subtropical North Atlantic and the tropics ( Giannini et al. 2013 ) and SST variability in the Mediterranean Sea (e.g., Fontaine et al. 2010 ) have also been shown to be important for providing skill ( Martin and Thorncroft 2014b ). Results from CMIP5 ( Goddard et al. 2013 ; Gaetani and Mohino 2013 ) show a nonnegligible contribution to the Sahelian rainfall skill from the external radiative forcing ( Fig. 10 ), whereas initialized hindcasts outperform empirical predictions based on persistence at longer lead times ( Gaetani and Mohino 2013 ; Martin et al. 2014 ).

The good performance of the ENSEMBLES ( García-Serrano et al. 2013 ) and CMIP5 ( Gaetani and Mohino 2013 ) decadal forecast systems in reproducing the relationship between the Sahel precipitation and its associated SST patterns encourages the promotion of improved decadal prediction systems in the future where the problems of SST biases are properly dealt with. This is only achievable in a context of a model development strategy across all communities that use climate models, as suggested by the WCRP.
Following the IPCC AR4, several studies were published on twenty-first-century rainfall scenarios over West Africa. The successful simulation of the Sahel rainfall requires the capture of several physical mechanisms with contributions that may have opposite signs ( Druyan 2011 ). The differential heating of land and ocean can strengthen the heat low in the Sahara and enhance the monsoon flow and thus rainfall ( Haarsma et al. 2005 ; Biasutti and Sobel 2009 ). Even in the absence of circulation changes, the warming-induced increase in atmospheric temperature would enhance precipitation in regions of moisture convergence such as the Sahel at the peak of the monsoon ( Held and Soden 2006 ; Seth et al. 2011 ). At the same time, warming could lead to increased stability and localized subsidence, in the future just as in the past ( Held et al. 2005 ; Seth et al. 2011 ). All these processes are likely to be relevant, but their influences may differ for different regions in North Africa, different times within the rainy season and, unfortunately, across different models (see, e.g., Giannini 2010 ).
Projections of summertime Sahel rainfall at the end of the twenty-first century by the CGCMs in CMIP3 and CMIP5 of the IPCC are shown in Figs. 11a and 11b for the emission scenarios SRES A1B and RCP4.5, respectively. Although the spread in rainfall projections is large at the end of the twenty-first century over the Sahel within both multimodel ensembles, there is evidence for a general tendency for slightly wetter conditions over central Sahel and drier conditions over Senegal, Mauritania, and southern Mali. A meridional dipole pattern of rainfall anomalies is also observed over the tropical Atlantic consisting of drier conditions over the Gulf of Guinea and wetter conditions north of 5°N. The multimodel agreement is better for the CMIP5 ( Fig. 11b ) than for the CMIP3 ensemble ( Fig. 11a ), and the results are more significant when the approach “one model, one vote” is used ( Monerie et al. 2013 ).

Another recent study of Roehrig et al. (2013) analyzes CMIP5 simulations along a range of time scales from interannual to decadal and future projections. CMIP5 climate change projections in surface air temperature and precipitation are found to be very similar to those obtained in CMIP3. Their results highlight a robust warming trend over the Sahel and an increase in rainfall in the eastern and a decrease in the western Sahel. However, there is a spread between models because of the deficiencies in simulating the Atlantic variability. In most of the models there is a systematic southward shift of the ITCZ.
The CMIP5 simulations reaffirm and strengthen the CMIP3 projections for changes in the seasonal cycle of Sahel rainfall (see, e.g., Biasutti and Sobel 2009 ; Biasutti 2013 ). A delay is projected on the start of the rainy season in the future throughout the Sahel, and especially over West Africa; instead, more abundant precipitation in the late rainy season is projected. There is robust agreement on the seasonality changes and the distinction between West Africa and the central Sahel in both CMIP3 and CMIP5. The projected increase in peak rainfall is consistent with a global pattern of enhanced seasonality of tropical rainfall ( Chou and Lan 2012 ; Chou et al. 2013 ). The drying at the onset of the rainy season might instead be a manifestation of the drying of the convective margins ( Neelin et al. 2003 ; Seth et al. 2011 , 2013 ).
Greenhouse gas (GHG) increases affect Sahel rainfall not only via the warming of global SST (slow response), but also via its direct effect on the temperature and energy fluxes of the land–atmosphere system (fast response; Patricola and Cook 2011 ; Giannini 2010 ). Using idealized CMIP5 simulations, Biasutti (2013) found that throughout the rainy season the slow response leads to less rain across the Sahel while the fast response forces wet anomalies that span the entire Sahel, although they are stronger in the east. Nevertheless, the fully coupled response, especially the change in seasonality, cannot be explained in terms of a simple linear superposition of the individual fast and slow responses. Biasutti (2013) speculates that the different phasing of the annual cycle over land and ocean interact to determine the projected delay of the Sahel rains in the coupled response.
Another proxy for drought in climate projections can be obtained by counting the annual number of dry days, which are defined as those when daily rainfall is less than 1 mm ( Frich et al. 2002 ; Vizy and Cook 2012 ). Vizy et al. (2013) indicate that current models underestimate the number of dry days along the Guinean coast and over the Congo basin, but conclude, on the basis of intermodel agreement and consistency across time periods, that there is a strong likelihood for fewer dry days over the Sahel of eastern Mali, Niger, Nigeria, and Chad and more dry days over Senegal and the far western Sahel. There is less agreement about how the number of dry days per year will change along the Guinean coast. These results are consistent with the seasonal mean changes discussed above but need to be reconciled with the expectations of Giannini et al. (2013) , who showed that Sahel rainfall recovery results from increases in mean daily accumulation rather than in frequency.
7. Summary, remaining questions, and future directions
The Sahel experienced starting in the 1970s one of the most dramatic droughts in the historical record, with consequences that have affected large areas and populations. The present paper reviewed studies on Sahel rainfall variability at time scales from interannual to decadal. The emphasis has been on the role of the oceanic anomalies, because according to the consensus view these are the most important contributors to rainfall variability in West Africa at the time scales of interest. Nevertheless, the influence of the land surface processes was also included as they are important contributors the West Africa rainfall variability. Future projections were also mentioned.
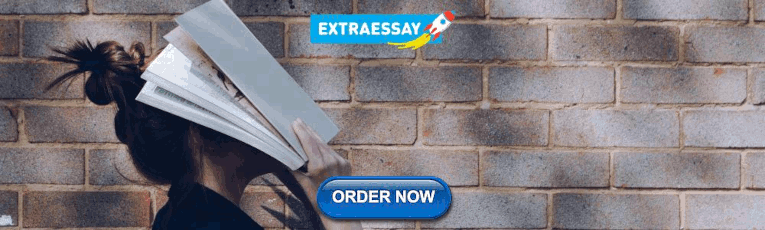
1) Interannual variability
In interannual time scales, a warming of the equatorial Atlantic and Pacific/Indian Oceans results in rainfall reduction over the Sahel. On the contrary, positive SST anomalies over the Mediterranean Sea tend to be associated with increased rainfall.
A dependence on time (nonstationarity) of these relationships has been found by recent studies ( Joly and Voldoire 2009 ; Rodríguez-Fonseca et al. 2011 ; Mohino et al. 2011b ; Losada et al. 2012 ). Before the 1970s positive SST anomalies over the Gulf of Guinea (Atlantic Niños) were associated with a dipole pattern of precipitation anomalies consisting of positive values along the coast of Guinea and negative ones over the Sahel ( Fig. 6 ). After the 1970s, a similar pattern of SST anomalies in the Gulf of Guinea appears to have local impacts but no significant remote impacts over the Sahel as SST anomalies in the Pacific seem to increase their influence in this region. Also the influence of SST anomalies in the Mediterranean Sea has increased in the last decades.
Current AGCMs generally capture these features. It has been argued that this time dependence is due to the changing configuration of tropical SST anomalies in different adjacent tropical oceans, which can interact either constructively or destructively according to time ( Losada et al. 2012 ). Figure 12 shows that the periods for which the Atlantic and Pacific may both strongly influence Sahelian rainfall present a multidecadal anomalous SST pattern resembling the positive and negative phases of the Atlantic multidecadal oscillation (AMO), respectively. Thus, a warmer or cooler climatological SSTs in the equatorial and tropical South Atlantic could play an active role.

In addition to SST variability, volcanic eruptions may influence the WAM rainfall some years ( Haywood et al. 2013 ),
2) Interdecadal variability
SST variability at decadal time scales provides the most important influence leading to drought over the Sahel. It has been shown that the global warming trend, positive phase of the interdecadal Pacific oscillation, and negative phase of the AMO lead to reductions in Sahel precipitation. State-of-the art CGCMs are able to reproduce the observed drying trend over the Sahel. However, models fail in obtaining the observed amplitude and neither generation of CMIP climate models reproduces the magnitude of the observed rainfall variability at decadal to multidecadal time scales over the twentieth century.
Observational evidence also indicates a strong decadal climate component in the Sahel and surrounding areas from the 1950s to the 2000s in vegetation cover and land use/land cover (LULC) change, as well as in aerosol types and spatial distributions (e.g., Rowell 2003 ; Xue et al. 2004a , b ; Lau and Kim 2007 ; Hastenrath and Polzin 2011 ). Vegetation dynamics, in particular, may contribute to the persistence of anomalies of the regional climate.
3) Mechanisms for SST impact
The mechanisms for SST impact operating at different climate scales, from interannual to decadal, are conceptually similar: the Atlantic interhemispheric gradients are related to changes in the position of the ITCZ and the warming of the Pacific tropical SSTs is related to anomalous equatorial atmospheric Rossby waves enhancing the subsidence over the Sahel.
A cooling in the equatorial Atlantic influences the large-scale dynamics, enhancing the meridional gradient in sea level pressure and strengthening the monsoon, while warming of the southern tropical Atlantic enhances the anomalous divergence over the Gulf of Guinea and the dipolar configuration of anomalous rainfall over Sahel. Anomalous Mediterranean Sea warm events are associated to a more intense monsoon with enhanced moisture convergence over the Sahel, which is advected by the climatological northerly flow. Moreover, an anomalous warming in the Pacific and Indian Oceans leads to reduced Sahel rainfall through an anomalous subsidence over the Sahel in association with an equatorial Rossby atmospheric wave.
4) Future projections
The agreement among future projections has improved from CMIP3 to CMIP5. Although rainfall projections for the Sahel have a large spread at the end of the twenty-first century, model projections exhibit a general tendency for slightly wetter conditions over central part and drier conditions over the western part. A meridional dipole pattern of rainfall anomalies is also obtained over the tropical Atlantic consisting of drier conditions over the Gulf of Guinea and wetter conditions north of 5°N. According to the projections, the start of the rainy season (onset) will be delayed in the future throughout the Sahel and especially over West Africa, with more abundant precipitation simulated during the late rainy season.
5) Forecasting
The skill of numerical forecasts has improved during the last decades. The increased attention to dynamical vegetation schemes has contributed to improvement. Prediction systems have evolved from seasonal to decadal forecasting. Current forecast systems show skill in their seasonal predictions of total rainfall amounts over West Africa, although this is generally limited to the Gulf of Guinea. Skill is improved when using multimodel predictions rather than single model ones. However, for harvesting purposes the timing of the rainy season is even more relevant than total rainfall amounts. Because of this, the seasonal forecasting community has lately focused on providing information beyond seasonal rainfall. Recent works show that there is useful skill to forecast the onset of the monsoon and that this skill can be related to SST anomalies in the tropical Atlantic in June. Moreover, the relevance of making actionable information available with a lead time of several years is recognized as a key planning horizon, making decadal prediction a new challenge for the scientific community. Significant correlation with the observations is found in some decadal predictions when simulating the relationship between the dominant WAM rainfall modes and their associated SST patterns, suggesting a potential for skillful decadal predictions in the future.
Significant shortcomings remain in the ability of numerical models to simulate some outstanding aspects of the tropical Atlantic climate and its variability.
Most contemporary coupled atmosphere–ocean (CGCMs) have important rainfall, wind, and SST biases in the tropical Atlantic. The simulations show too weak trade winds, a spurious ITCZ south of the equator, and too warm eastern tropical SSTs, compromising the successful representation of WAM and its response to tropical Atlantic variability.
CGCMs have difficulties in capturing the relationships among Pacific SSTs and WAM rainfall anomalies during the developing phase of an El Niño (La Niña) event.
There are large differences among models in the intensity and variance of simulated precipitation and the majority of CGCMs fail to produce proper intensities of the African easterly jet and the tropical easterly jet.
RCMs capture the northward jump of the precipitation band that represents the monsoon onset over Sahelian Africa, which GCMs fail to simulate. However, the jump in the RCMs occurs earlier than observed, suggesting serious model limitations for predicting the timing of monsoon onset.
It is very important to emphasize that over West Africa, RCMs will not necessarily provide better results than GCMs because both modeling systems show a number of similar biases that are still unresolved. The impact community, in particular, must exercise caution in the interpretation of results from impact models forced by GCM and/or RCM models. At this point in time, such an exercise should be considered more as a scientific enquiry than an operational forecast.
Since forecasts are sensitive to the quality of the data used to initialize the model, an improvement of the initial conditions used over the equatorial and extratropical Atlantic as well as over land is just as essential to improve the forecast quality as the model improvement is.
The role of the Indian Ocean in forcing interannual rainfall anomalies over West Africa is not clear as some papers have reached contradictory conclusions.
The reasons for the nonstationary impact of tropical SST anomalies in different basins are not clear at the present time. There are at two competing hypotheses that require further validation: statistical artifacts due to sampling errors and modulations by changes in the ocean climatology, which introduce nonlinearities in the system and, thus, different impacts.
Despite the general agreement that the long-term Sahel drought was mainly driven by SST variability and amplified by land surface processes, the details as to which basin or SST pattern lead this drought are still debated. Some works suggest that the warming of the tropical SSTs in the Indian, Pacific, and/or Atlantic Ocean basins were the dominant factors, while others place a more fundamental role on the interhemispheric north–south SST gradient in the Atlantic.
Another key point still under debate is the attribution of the long-term Sahel drought to anthropogenic factors, as greenhouse gases or anthropogenic aerosols. On the one hand, some works suggest that these factors played little or no role in the twentieth-century Sahel drought. On the other hand, other studies argue they were the main driver of the drought.
There is still a substantial spread in rainfall projections, which is large at the end of the twenty-first century over the Sahel.
What are the key model components to be improved for a higher skill of drought prediction over the Sahel? Should radiative impacts of natural aerosols from the continent be considered in reference to the systematic errors of CGCMs over the Atlantic, both in the subtropics and the tropics? The answers to these questions may require the realization of field campaigns in the eastern tropical Atlantic.
Could land surface processes become the primarily responsible for drought over West Africa in change scenarios?
One of the most dramatic and immediate impacts of climate variation is on disease, especially the vector-borne diseases that disproportionally affect the poorest populations in West Africa ( Hoshen and Morse 2004 ; Caminade et al. 2011 ). To assist with their short-term management and make projections of their future likely impacts, the project Quantifying Weather and Climate Impacts on Health in Developing Countries (QWECI) has developed and tested the methods and technology required for an integrated decision support framework for the quantification and prediction of climate and weather on health impacts in Africa. In particular, while there is useful skill in reproducing global and regional temperatures ( Doblas-Reyes et al. 2013 ; Guemas et al. 2015 ), the reduced skill in forecasting WAM rainfall anomalies ( Doblas-Reyes et al. 2013 ) prevents its use in driving health-impact models (which also require rainfall estimates) on decadal time scales ( MacLeod et al. 2012 ).
New European projects are being launched to enhance predictability of this region. For example, the Seasonal-to-Decadal Climate Prediction for the Improvement of European Climate Services (SPECS) EU project ( http://www.specs-fp7.eu/ ), which started in 2012, produces quasi-operational and actionable local climate information at seasonal-to-decadal time scales over the longest possible observational period over land, focusing on Europe, Africa, and South America. The aim is to provide information with improved forecast quality, focusing on extreme climate events to enhanced communication and services for wide range of public and private stakeholders. Also, EU project Enhancing Prediction of Tropical Atlantic Climate and Its Impacts (PREFACE), which started in November 2013, aims to reduce uncertainties in our knowledge of the tropical Atlantic climate, improving climate prediction and the quantification of climate change impacts in the region. In turn, the recently launched EU Dynamics–Aerosol–Chemistry–Cloud Interactions in West Africa (DACCIWA) project, which started in December 2013, aims at improving our understanding of interactions between emissions, clouds, radiation, precipitation, and regional circulation over West Africa, which will reduce model uncertainties in climate predictions over the area. The observational datasets, acquired within AMMA and more recent programs such as Fennec ( Washington et al. 2012 ) are the backbone of modern research efforts.
Acknowledgments
Belen Rodríguez-Fonseca, Elsa Mohino, and C. Roberto Mechoso wrote and organized this review. We thank additional contributions by the other authors as follows. Section 1 : William Lau, Edward K. Vizy, Kerry Cook, and Serge Janicot; section 2a : Teresa Losada and Irene Polo; section 2b : Marco Gaetani and Bernard Fontaine; section 2c : David P. Rowell; section 2d : Juergen Bader; section 2e : Aurore Voldoire and David P. Rowell; section 3b : Yongkang Xue and Fred Kucharski; section 4 : Alberto Arribas, Andrew Colman, and M. Vellinga; section 5 : Javier Garcia-Serrano, Francisco Doblas Reyes, Lisa Goddard, and Marco Gaetani; and section 6 : Cyril Caminade, Michela Biasutti, Leonard Druyan, Edward K. Vizy, and Kerry Cook.
This work was supported by Spanish projects MINECO CGL2011-13564-E and GL2012-38923-C02-01. Support from the U.S. National Science Foundation (Awards SES-1048946, ATM-1036604, AGS-1041477, and AGS-1115506) is gratefully acknowledged. Also gratefully acknowledged are the GCM modeling groups, the Program for Climate Model Diagnosis and Intercomparison (PCMDI), and the World Climate Research Programme’s Working Group on Coupled Modeling (WGCM) for their roles in making available the WCRP CMIP5 multimodel dataset. Support of this dataset is provided by the Office of Science, U.S. Department of Energy (DOE). We acknowledge the EU QWECI, PREFACE, and SPECS projects both funded by the European Commission’s Seventh Framework Research Programme under Grant Agreements 243964, 603521, and 308378 respectively. Based on a French initiative, AMMA was built by an international scientific group and is currently funded by a large number of agencies, especially from France, the United Kingdom, the United States, and Africa. It has been the beneficiary of a major financial contribution from the European Community’s Sixth Framework Research Programme. The authors are grateful to Ashlynn Fiss for her help in improving this manuscript.
AMS Publications

Get Involved with AMS
Affiliate sites.
Email : [email protected]
Phone : 617-227-2425
Fax : 617-742-8718
Headquarters:
45 Beacon Street
Boston, MA 02108-3693
1200 New York Ave NW
Washington, DC 200005-3928
- Privacy Policy & Disclaimer
- Get Adobe Acrobat Reader
- © 2024 American Meteorological Society
- [66.249.64.20|185.39.149.46]
- 185.39.149.46
Character limit 500 /500
Analysis: Understanding the Sahel drought
Scientists say that the current drought in the Sahel began as far back the 1960s.

Between the vast Sahara Desert and the dense foliage of the African Rainforest is a band of semi-arid grassland. Known as the Sahel, this hardy landscape is one of Africa’s most productive crop regions.
But the crops in the Sahel are grown close to their limits of tolerance, and rely on natural rainfall. This means that even small changes to the amount of rain can have disastrous effects.
Keep reading
Evacuation orders issued as wildfire grows near canada’s alberta oil patch, energy summit seeks to curb cooking habits that kill millions every year, thousands evacuate as wildfire grows ‘dramatically’ in western canada, guardians of the glaciers – life alongside pakistan’s vanishing ice.
Unfortunately, this is a region which often suffers highly erratic rainfall.
Droughts in the Sahel don’t last a month or a year, they last for decades. And these long droughts have occurred regularly over the past 12,000 years.
The region is in one of these droughts now – scientists say it started in the 1960s.

Between the 1950s and 1960s, there was plenty of rain across the Sahel, but just before 1970, a new drought started.
With the exception of a period of five years, all years since have seen a deficiency of rain.
The most severe drought was in the 1980s and, since then, levels of rainfall have recovered a little. However, rainfall remains significantly below the average for the past century.
The rain across the Sahel spreads up from the south during the summer months. The onset is usually in June, with the majority of the rain falling between July and September.
Overall, the amount of rain received in the 2011 monsoon was near normal, but this doesn’t tell the whole story: the rains were patchy and irregular, slow to get going, and retreated very quickly. This left many regions facing water and food shortages.
Reasons for the drought
Compiling reasons for the drought is difficult, but what is known is that the rainfall across the Sahel is driven by three factors:
- Tropical convection: the huge towering thunderstorms that build quickly in tropical places.
- The West African Monsoon: rains which starts to spread across the Sahel in June.
- El Niño: the effects of the warming of the Pacific, which also affects the weather around the globe.
But there is little understanding of how these factors link together and how they are affected by the changing climate.
In the 1970s it was widely believed that the drought was caused by the farmers in the regions, blaming them for the degradation of the land and the desertification. However, subsequent studies have disproved this idea.
Currently, the most convincing theory is that the recent drought could be due to the change in temperature of the surrounding oceans.
At the end of the 1900s, the south Atlantic and the Indian Ocean warmed quickly, which reduced the difference in temperature between the land and the sea. This may have caused the monsoon to weaken and the thunderstorms to remain to the south.
When the rains do fail, there is concern that this could trigger a knock-on effect in the years to follow.
Less rain means less vegetation, which will lead to a change in colour of the ground. Instead of lush fields of plants colouring the earth in a deep green, which absorbs sunlight, the ground instead would be a lighter, barren beige, which reflects more sunlight.
This, in turn, would mean the temperature difference between the land and the sea was diminished, and would lead to further weakening of the monsoon.
To the future
It is most important to predict what will happen in the future, but currently it is almost impossible.
The difficulty is due mostly to the scant availabilty of historical data from across the region. Therefore, discovering how the climate is changing is an uphill struggle.
The current thought is that the Horn of Africa will become wetter, but what will happen across the Sahel is unknown: will it become wetter or drier? No one can be sure.
What is expected is that the rainfall will become more sporadic, bringing an increase in the number of both droughts and floods across the region.
The 2012 forecast
The monsoon forecast for the Sahel region has just been issued by the African Centre of Meteorological Applications for development (ACMAD). This is the consensus forecast for the region, drawing together forecasts from many different countries.
The forecast focuses on the months of July to September, when the majority of the rain falls across the region.
This year, the forecast suggests that the majority of the Sahel will experience average rainfall, or even above average in some locations.
The exception is the Western Sahel, which is only estimated to receive 70 – 90 per cent of their annual rainfall, and here the onset of the rains is likely to be delayed.
This means that Senegal, southeastern Mauritania, Western Mali and Gambia are all likely to face severe disruptions.
Farmers in these regions are being encouraged to prioritise crops and sow water-stress resistant seeds.
What happens next
Clearly more research is desperately needed – because even small changes in rainfall, both timings and amounts, can have dire impacts on the people who live in the Sahel.
To try to ensure that national governments have access to the best scientific information available, Africa Climate Exchange (Afclix) has recently been launched by a team led by the University of Reading’s Dr Rosalind Cornforth.
Not only does the site help ensure that policy makers have direct access to the latest forecasts, but Afclix helps different research teams with varying expertise connect with each other, enabling collaborations and cross-disciplinary research.
The more studies carried out, the greater the chance there is of discovering the secret of the Sahel rains.
As the knowledge grows, the forecasts will improve and the impacts on the local population can be minimised.
Steff Gaulter is Al Jazeera’s senior meteorologist. Follow her on Twitter: @WeatherSteff
Daily Press Briefing by the Office of the Spokesperson for the Secretary-General
The following is a near-verbatim transcript of today’s noon briefing by Farhan Haq, Deputy Spokesman for the Secretary-General.
All right, good afternoon, everyone, and happy Friday.
** Secretary-General’s Travels
In Nairobi, the Secretary-General spoke today at the United Nations Civil Society Conference in Support of the Summit of the Future.
He said that, whether on climate, gender equality or peace, he has witnessed the enormous impact of civil society in every corner of the world . He encouraged the representatives — most young people — to continue working with the United Nations to build a better world.
As outlined in his Our Common Agenda report, Mr. [António] Guterres said we need a vision for a more inclusive, networked and effective multilateralism — one where the contributions of civil society are recognised as central, not a token or an afterthought. He said: “We won’t give up in the struggle for peace, justice and human rights, and I know you won’t either. My best hope for the future is you.”
In remarks to the press, the Secretary-General extended his deepest condolences to all those affected by the devastating floods that have swept through Kenya and neighbouring countries. The United Nations stands in solidarity with the Kenyan people, he said. He added that we will continue working hand in hand to support Government relief efforts in any way that we can.
The Secretary-General reiterated his appeal for the Government of Israel and the leadership of Hamas to demonstrate political courage and spare no effort to reach agreement to stop the bloodshed — and to free the hostages.
He said that we are actively engaging with all involved for the resumption of the entry of life-saving supplies — including desperately needed fuel — through the Rafah and Kerem Shalom crossings. But he warned that a massive ground attack in Rafah would lead to an epic humanitarian disaster and pull the plug on our efforts to support people as famine looms.
In a bilateral meeting with the President of Kenya, William Ruto, the Secretary-General expressed his condolences for the loss of life and damage caused by recent flooding. He also saluted Kenya’s leadership of the Multinational Security Support Mission that is so desperately needed for Haiti.
On the sidelines of the conference, the Secretary-General will meet with the co-facilitators of the Summit of the Future, the Permanent Representatives of Namibia and Germany.
After Nairobi, the Secretary-General will be heading to Kuwait, Oman and Bahrain. On Sunday, 12 May, he will arrive in Kuwait City early in the morning for an official visit. While there, he is expected to hold meetings with His Highness, Sheikh Meshal Al-Ahmad Al-Jaber Al-Sabah, Amir of the State of Kuwait. Discussions will focus on the situation in the region and beyond.
The second stop will be on Monday, 13 May, where he will be heading to Oman, for more discussions. In Muscat, he will meet with His Majesty Sultan Haitham Bin Tarik.
From there, the Secretary-General will travel to Manama, in Bahrain, where the Arab League Summit is taking place. He will deliver remarks to the summit.
In Manama, the Secretary-General will hold discussions with leaders attending the summit.
We will keep you updated during each leg of his visit.
** Occupied Palestinian Territory
The Office for the Coordination of Humanitarian Affairs (OCHA) says that ground incursions and heavy fighting continue to be reported in eastern Rafah, including around the Kerem Shalom and Rafah crossings.
The Director-General of the World Health Organization (WHO), Dr. Tedros Adhanom Ghebreyesus, said that a WHO staff member, his spouse and their child were injured two days ago in Rafah when their home was destroyed due to an air strike nearby. The staff member’s 7-year-old niece was killed. Dr. Tedros called for the protection of all civilians and humanitarian workers.
The UN Relief and Works Agency, UNRWA, reports that more than 142,000 people already displaced from Rafah are facing dire shortages of food, water, shelter and sanitation services.
Humanitarian partners working on the health response in Gaza warn that with fuel supplies dwindling, five hospitals and five field hospitals, 17 primary health-care centres, nearly two dozen medical points and 28 ambulances will only be able to sustain their operations for less than 48 hours.
Meanwhile, eight bakeries supported by the World Food Programme in southern Gaza have already ceased operations, and four others will soon run out of fuel and supplies. The four operational bakeries in northern Gaza have one week of supplies available to make bread.
Humanitarian missions to northern Gaza continue to face significant access constraints. As of yesterday, only 9 of 32 aid missions to northern Gaza this month have been facilitated by Israeli authorities.
The Under-Secretary-General for Humanitarian Affairs, Martin Griffiths, said in a social media post yesterday that civilians in Gaza are being starved and killed as the humanitarian community is prevented from helping them.
He warned that the closure of the crossings means no fuel, trucks, generators, water or electricity — and no movement of people or goods.
On Thursday evening, Israeli residents set fire twice to the perimeter of the UNRWA headquarters in occupied East Jerusalem. This took place while UNRWA and other UN agencies’ staff were on the compound.
While there were no casualties among our staff, the fire caused extensive damage to the outdoor areas.
A crowd accompanied by armed men were witnessed outside the compound chanting, “Burn down the United Nations”.
This is an outrageous development, Commissioner-General Philippe Lazzarini said. Once again, the lives of UN staff were at a serious risk.
In light of this second appalling incident in less than a week, he has taken the decision to close down the compound until proper security is restored.
He said it is the responsibility of the State of Israel as an occupying Power to ensure that United Nations personnel and facilities are always protected.
UN staff, premises and operations should be protected at all times in line with international law.
The Secretary-General condemned the attack. Targeting aid workers and humanitarian assets is unacceptable and must stop, he said.
The Office for the Coordination of Humanitarian Affairs (OCHA) welcomes the Government of Syria’s authorization today for the continued use of the Bab al-Salam and al-Rai crossing points from Türkiye, for the United Nations to continue delivering humanitarian assistance to people in need in north-west Syria, through August 13 th .
The cross-border operation from Türkiye — which also utilizes the Bab al-Hawa crossing — is a lifeline for aid to north-west Syria, where millions of people are in need of humanitarian assistance, including food, nutrition, health, shelter, protection, education and other critical support.
This year, we and our partners are appealing for just over $4 billion to assist more than 10 million people throughout Syria. But our ability to respond continues to be constrained by reductions in funding, with only 6 per cent of the funding required — some $224 million — received to date.
On Haiti, our humanitarian colleagues warn that the prevalence of sexual and gender-based violence has reached an alarming level in the country. According to a joint report by the Government and our humanitarian partners released today, the number of gender-based violence survivors was five times higher in March than it was in January and February combined.
Some three quarters of the cases concern sexual violence, with 94 per cent of survivors being girls and women, while 78 per cent of survivors are internally displaced people.
The number of cases of gender-based violence committed by members of armed groups represents 72 per cent of reported incidents.
Humanitarian organizations continue to support survivors, with 90 per cent of survivors having received psychosocial support and 25 per cent of rape victims receiving medical care within 72 hours following the incident. More than 1,300 dignity kits have been distributed to displaced people living in sites.
Despite the increasing number of gender-based violence cases, humanitarian organizations have only received 7 per cent of the funding required for prevention and to support survivors. We urgently need additional resources to strengthen the response and provide legal assistance, medical care, psychosocial support and livelihood means for survivors.
The Office for the Coordination of Humanitarian Affairs tells us that Chad continues to face a protracted humanitarian crisis, with 6 million out of 18.8 million people in need of humanitarian assistance across the country.
The food security and nutrition situation is being exacerbated by climate change, insecurity and the rise in food and fuel prices.
According to a UN-backed analysis, 2.4 million people are currently food insecure in the country — 300,000 of whom are severely food insecure. This number could grow during the lean season to 3.3 million, if no substantive assistance is provided.
Since March, the country is also facing torrential rains and floods in the south of the country.
The country has also welcomed more than half a million refugees since the start of the conflict in neighbouring Sudan last year. This has significantly strained the humanitarian situation in the east of the country, and attacks by non-State armed groups in the Lake Chad Basin area are driving further displacement.
The $1.1 billion Humanitarian Needs and Response Plan is just 6.6 per cent funded with nearly $74 million received.
The Emergency Relief Coordinator, Martin Griffiths, allocated $15 million from the Central Emergency Response Fund (CERF) as part of a larger allocation in February this year to support underfunded emergencies.
** Zimbabwe
Turning to Zimbabwe, we and our partners launched yesterday a Flash Appeal seeking $429 million to support 3.1 million people.
This appeal comes following an El Niño-induced drought that has affected the country since December of 2023; it will complement the Government’s response to the national drought disaster.
In Zimbabwe, El Niño events have been associated with prolonged dry spells, reduced rainfall and increased temperatures.
An estimated 6 million people are expected to be food insecure during the lean season.
The drought is worsening water shortages, potentially affecting 2.6 million people.
In view of the worsening situation, the Emergency Relief Coordinator, Martin Griffiths, released $13.5 million last month from the Central Emergency Response Fund to address the El Niño-induced drought in Southern Africa. Of this amount, $3 million of this allocation went to Zimbabwe.
The Assistant Secretary-General for Humanitarian Affairs, Joyce Msuya, will visit Kuwait this week, as the country hosts a humanitarian conference on Gaza.
The one-day event on Sunday, 12 May, will bring together UN agencies and international, regional and local aid organizations in Kuwait to explore urgent measures to stem the spiralling humanitarian catastrophe in Gaza.
Ms. Msuya will speak at the opening of the conference, which is being organized by the International Islamic Charitable Organization, in partnership with OCHA and under the patronage of the Prime Minister of Kuwait.
Today in Kyiv, the UN team, alongside the Governments of Germany and Ukraine, hosted a forum focused on Ukraine’s recovery from the ravages of war. The event, a precursor to the forthcoming Ukrainian Recovery Conference in Berlin next month, saw nearly 500 participants, including government officials, local authorities, civil society, community leaders and national and international development partners.
A key focus of the discussions was ensuring that recovery efforts are inclusive and address the needs of marginalized groups, including women, people with disabilities, the Roma and the LGBTQI+ community.
The UN team notes that Russia’s ongoing invasion has caused immense human suffering and widespread devastation, leading to dire humanitarian needs. Last year alone, the UN helped rebuild nearly 1,000 schools, provided primary health care to more than 5 million women and children, installed new heating, water, and energy infrastructure for 6 million people and supported more than 2,100 small and medium businesses.
** Peacebuilding Commission
The Chair of the Peacebuilding Commission (PBC), Sérgio França Danese of Brazil, will undertake a visit to São Tomé and Príncipe from 13 to 15 May at the invitation of the Prime Minister, Patrice Trovoada.
The President of the Commission of the Economic Community of Central African States (ECCAS), Gilberto Da Piedade Verissimo; the Special Representative of the Secretary-General for Central Africa and Head of the United Nations Regional Office for Central Africa, Abdou Abarry; and the United Nations Assistant Secretary-General of the Peacebuilding Support, Elizabeth Spehar, will accompany the Chair.
The mission is intended to mobilize support to São Tomé and Príncipe in consolidating peace and stability, notably reforming its justice and security sectors.
** Senior Personnel Appointment
We have a senior personnel appointment. Today, the Secretary-General is appointing Barrie Freeman of the United States as his new Deputy Special Representative for West Africa and the Sahel (UNOWAS).
Ms. Freeman succeeds Giovanie Biha of Burundi, to whom the Secretary-General is grateful for her accomplishments in West Africa and the Sahel, including her role in advancing implementation of the UN Integrated Strategy for the Sahel. The Secretary-General also thanks Khassim Diagne of Senegal, who has been providing steadfast support to UNOWAS in the interim.
Ms. Freeman brings to the position over 30 years of wide-ranging experience in international peace and security. She has served since 2021 as Deputy Special Representative for the United Nations Interim Administration Mission in Kosovo (UNMIK). Before that, she was Deputy Head and Political Director in the Peacebuilding Support Office of the Department of Political and Peacebuilding Affairs from 2018 to 2021.
There is more online.
** International Days
Today is the International Day of Argania. As you may know, the argan tree is a native species of the sub-Saharan region of Morocco.
Tomorrow is World Migration Bird Day. This Day shines a spotlight on the relationship between migratory birds and insects amidst alarming declines for both.
And on Sunday, we mark the International Day of Plant Health. It’s a reminder that we depend on plants for 80 per cent of the food we eat and 98 per cent of the oxygen we breathe.
** Financial Contribution
And it’s Friday, and it’s nearly lunchtime.
If we were lucky enough to have lunch in the latest Member State to pay its dues, we might be treated to, amongst many other foods, Kuy teav, Lap Khmer or Samlar kari. Anyone willing to guess what that nation is? It is Cambodia.
We thank our friends in Phnom Penh for Cambodia’s payment, and we have reached 108 fully paid-up nations.
** Questions and Answers
Deputy Spokesman : Yes. Dezhi and then Amelie.
Question : A couple of questions. Today, the GA vote adopted the resolution, and in that resolution, it asked Secretary-General to take necessary measures to implement the resolution, which grants many rights to the State of Palestine. What measures can the Secretary-General do to implement that resolution? Will he refer to Security Council to restart the negotiation of the admission again? And what measures?
Deputy Spokesman : Well, regarding that, in accordance with our standard procedure, the Secretary-General and the Secretariat will take the measures necessary to implement the resolution, as requested by the Assembly. [cross talk]
Question : So, what are the necessary measures?
Deputy Spokesman : We will, first now that it’s passed, we will study the resolution, including its annexes, and see how to go forward in that. But we will then proceed with that.
Question : And one thing, today the Israeli Ambassador shredded the UN Charter; said that’s what people did in that, in that hall. What is the reaction of the Secretary-General on that image? Does he think they can do that? [cross talk]
Deputy Spokesman : We’ve told you before that we don’t comment on the various remarks made by different ambassadors. And the theatrics, both in the past and the present, are things that are part and parcel of the presentations the Member States make. We don’t comment on those, but regarding the Charter, obviously, this is an organization that is premised on respect with the UN Charter, and all of the Member States have pledged to uphold the UN Charter, and we expect them to fulfil that obligation.
Question : And do you feel uncomfortable to see that image in the TV?
Deputy Spokesman : I’ve been around here a long time. I’ve seen a lot. Look… [cross talk] All I can tell you, look, that was a Charter. There’s many charters. [pulls out a copy of the United Nations Charter] There’s one right here. The Charter is intact. Its ideals are intact.
Correspondent : You resurrected it.
Deputy Spokesman : What?
Correspondent : You resurrected it. [laughing] Good job.
Deputy Spokesman : The Charter is something that will stay with us. And like I said, it’s the founding document of this organization. As long as this organization exists, the Charter exists.
Question : Now that… Why I asked that question, because we saw the attack on UNRWA, and we know it might be some people who hated UNRWA, who had opinions on UN, they did that. But don’t you think this kind of behaviour by the Israeli Ambassador, actually… how to say that? Infused this kind of behaviour, infused this attack for UNRWA, for those people who stopped the trucks, aid trucks, to cross the Kerem Shalom border crossings?
Deputy Spokesman : Well, those are separate things. Obviously, a piece of paper is a piece of paper and theatrics are theatrics… [cross talk]
Question : But those…
Deputy Spokesman : We are against, we are clearly and strictly against threats made on our personnel and our facilities. Those are irresponsible, they’re wrong, they have to stop.
Question : But it’s not connected, you think?
Deputy Spokesman : We have seen so much. You can go over the history of the UN and all the speeches that happened. You know, different moments when people use different props. Their own shoes, their… the various resolutions of the Security Council that got crumpled up by different people; we’ve seen it all. We rise above that. This is an organization of diplomacy, and we encourage diplomacy.
Question : Thanks, Farhan. A completely different topic. On Somalia, the Government of Somalia has sent a letter to the SG and the Security Council requesting the termination of UNSOM (UN Mission in Somalia). Do you have any comment on that? Do you think UNSOM has done enough in Somalia that they could end their mission?
Deputy Spokesman : Well, the thing about UN missions is that we do not plan to stay forever in any country. But when we withdraw, we want to do it as part of dialogue with the country, with the Security Council and others on how to do it. Decisions on the mandate of UNSOM, the UN Mission in Somalia, have to be taken by the Security Council. So really, this matter rests in their hands, and we will accept what the Security Council does, but we will work in good faith with all Member States in terms of what’s expected if there is to be a drawdown of a UN mission. And I might add, if a political mission or a peacekeeping mission leaves a country, that doesn’t mean the end of the UN presence. We have country teams, we have other ways of assisting, and we would continue to assist the people of Somalia.
Question : But I mean, we’ve seen in recent months, I mean, in a year, many different Governments out of the blue requesting the UN missions, whether political missions or peacekeeping mission, to leave. So, are you worried about this tendency?
Deputy Spokesman : There are different moments, if you look at the history of the UN, when political missions, peacekeeping missions either grow or they ebb. We are well aware of that process. And what we try to do is make sure that whenever we have to leave anywhere, it’s done in a responsible manner so that we can continue to serve the people of that place — in this case, Somalia.
Yes, Ibtisam?
Question : Farhan, first on the resolution that was adopted today, do you have any comments on that?
Deputy Spokesman : Well, what I can tell you, our basic comment is that it’s clear, including from the resolution, that the State of Palestine was not granted a new status within the United Nations, nor has it been admitted to membership in this Organization. It is also clear that it remains an Observer State and does not have the same status nor standing as a Member State. At the same time, of course, the annex provides for additional rights and privileges of participation of the State of Palestine, without prejudice to its existing rights and privileges, and we will follow what the General Assembly has agreed to regarding that.
Question : Yeah. Well, my question is whether you have a comment on the resolution? Do you welcome it? Do you think it’s a right step in the right direction, giving the fact… in light of your resolutions?
Deputy Spokesman : Well, certainly, this is a new resolution for us to follow, and we welcome the fact that a wide membership of the General Assembly has agreed to this step.
Question : I have another question on attack on your premises, UNRWA premises of the UN in Jerusalem. As you mentioned in the statement you read that this is the… it is happening for the second time in the same week. And when I asked Stéphane [Dujarric] about it, the last week — I’m sorry, not last week; earlier this week — he said that because of the first attack, he said that you are communicating with the Israelis on this issue, but it seems to be that this communication didn’t bear fruits in the way that your premise was attacked again. And this time, bigger attack that led to a burn down of some parts of the building. And I guess my question here, again, how concerned are you about the safety of your staff in Jerusalem and about the fact that the Israeli Government is not doing what they need to do as a host country in protecting your premises?
Deputy Spokesman : We’re very concerned, and you can see how concerned we are from the fact that Mr. Lazzarini took the decision not to bring any staff into the compound until conditions there are safe and until the safety can be guaranteed by the relevant authorities. We have reminded Israel and will continue to remind Israel of its obligations as a host country in occupied East Jerusalem.
And with that… Yes, one more.
Question : I came late. I didn’t want to jump in front of anybody else. A question on numbers. The Director of UNICEF (United Nations Children’s Fund), Catherine Russell, had indicated in mid-March she was citing Hamas’ health ministry numbers. Thirteen thousand children had died. That was in mid-March in Gaza. The UN released totals yesterday, showing that less than 8,000 children have died in Gaza as a result of the war, citing those… that same Hamas’ health industry. Can you clarify the process for how those numbers were revised?
Deputy Spokesman : Yeah, the revisions are taken… you know, of course, in the fog of war, it’s difficult to come up with numbers. We get numbers from different sources on the ground, and then we try to crosscheck them. As we crosscheck them, we update the numbers, and we’ll continue to do that as that progresses.
Question : I mean, that’s… it’s almost half. I mean, it’s pretty significant.
Deputy Spokesman : Numbers get adjusted many times over the course of a conflict. Once a conflict is done, we’ll have the most accurate figures. But we’re just going with what we can absolutely confirm, which will always be the low end of what the numbers are.
Question : So, can people consider those numbers reliable having been so off in this occasion?
Deputy Spokesman : Well, you can consider them reliable from the fact that we’re continually checking them. We’ll continue to do that over the course of the war. But the numbers, you know, ultimately have to be regularly checked so that we can be sure that what we’re putting out is valid.
Okay. Anything else? If not, I yield a floor to the real star of today, the Spokesperson for the President of General Assembly, Monica Grayley.
Facebook Twitter Email Print LinkedIn
- Media Resources
- 2030 Agenda
Migration and Migrants: Regional Dimensions and Developments
World migration report 2024: chapter 3.
Migration in Africa involves large numbers of international migrants moving both within and from the region. 4 As shown in Figure 1, most international migration occurs within the region. The latest available international migrant stock data (2020) 5 show that around 21 million Africans were living in another African country, a significant increase from 2015, when around 18 million Africans were estimated to be living outside of their country of origin but within the region. The number of Africans living in different regions also grew during the same period, from around 17 million in 2015 to over 19.5 million in 2020.
Figure 1 shows that since 2000, international migration within the African region has increased significantly. Since1990, the number of African migrants living outside of the region has more than doubled, with the growth in Europe most pronounced. In 2020, most African-born migrants living outside the region were residing in Europe (11 million), Asia (nearly 5 million) and Northern America (around 3 million).
One of the most striking aspects to note about international migrants in Africa, as shown in Figure 1, is the small number of migrants who were born outside of the region and have since moved there. From 2015 to 2020, the number of migrants born outside the region remained virtually unchanged (around 2 million), most of whom were from Asia and Europe.

An official website of the United States government.
If you want to request a wider IP range, first request access for your current IP, and then use the "Site Feedback" button found in the lower left-hand side to make the request.
Drought in the Sahel
- Published: August 1999
- Volume 48 , pages 299–311, ( 1999 )
Cite this article
- C.T. Agnew 1 &
- A. Chappell 2
1168 Accesses
82 Citations
52 Altmetric
Explore all metrics
The Sahel region of West Africa is well known as a region of environmental degradation. The reported incidence of desertification has been challenged but persistent and widespread drought is still widely accepted. Drought, defined solely as a function of rainfall, is believed to have commenced in the early 1970s and continued through to the present. It is usually defined as a meteorological phenomenon and standardised rainfall anomalies are employed to indicate the severity of negative departures from the ‘norm’. There are several difficulties with this approach. The period of standardising rainfall has changed from 1931–1960 to 1961–1990 but the impacts on drought occurrence have not been fully determined. The spatial aggregation of rainfall anomalies may mask important local variation and the purely statistical approach to defining drought takes little account of human impact. The first two issues, averaging period and spatial aggregation, are investigated through an analysis of rainfalls in Continental Sahel (Bukina Faso, Mali and Niger). A new classification of drought classes is suggested. Despite the clear evidence of negative rainfall anomalies for rainfalls aggregated across the Sahel region, it is found that the averaging period has a significant impact on our perceptions of the occurrence of what can be considered to be meteorological drought according to the definition employed and that there is significant spatial variation.
This is a preview of subscription content, log in via an institution to check access.
Access this article
Price includes VAT (Russian Federation)
Instant access to the full article PDF.
Rent this article via DeepDyve
Institutional subscriptions
Similar content being viewed by others
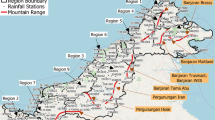
Space–time heterogeneity of drought characteristics in Sabah and Sarawak, East Malaysia: implications for developing effective drought monitoring and mitigation strategies
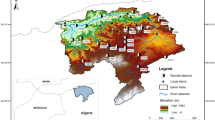
Spatiotemporal analysis of meteorological drought in a Mediterranean dry land: case of the Cheliff basin–Algeria
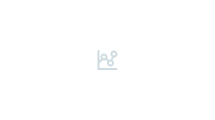
Extreme Historical Droughts in the South-Eastern Alps — Analyses Based on Standardised Precipitation Index
Agnew C.T., 1982: Water availability and the development of rainfed agriculture in S.W. Niger. Transactions of the Institute of British Geographers 7 : 419–457
Article Google Scholar
Agnew C.T., 1989: Sahel drought, meteorological or agricultural? International Journal of Climatology 9 : 371–382.
Google Scholar
Agnew C.T., 1990: Spatial aspects of drought in the Sahel. Journal of Arid Environments 18 : 279–293.
Agnew C.T., 1991a: Evaluation of a soil water balance model for the analysis of agricultural drought in the Sahel. In: Sivakumar M.V.K., Wallace J.S., Renard C. and Giroux C. (eds), Soil Water Balance in the Sudano-Sahelian Zone , IAHS Publication 199, Wallingford, pp. 583–592.
Agnew C.T., 1991b: Disaster in the arid realm. In: Slater F. (ed.), Societies: Choices and Environments . Collins Educational, London, pp. 56–79.
Agnew C.T., 1995: Desertification, drought and development in the Sahel. In: Binns A. (ed.), People and Environment in Africa . J. Wiley & Sons, Chichester, pp. 137–149.
Agnew C.T. and Warren A, 1996: A framework for tackling drought and land degradation. Journal of Arid Environments 33 : 309–320.
Agnew C.T. & Anderson E., 1992: Water Resources in the Arid Realm . Routledge, London.
Agnew C.T. and Chappell A., 2000: Desiccation in the Sahel. In McLaren S. and Kniveton D. (eds), Linking Climate Change to Land Surface Change: Proceedings of TIBG-RGS Conference , Leicester, January 1999. Kluwer Academic Publishers, Dordrecht (in press).
Ahlcrona E., 1988: The impact of climate and man on land transformation in central Sudan. Applications of Remote Sensing, Meddelanden fran Lunds Universitets Geografiska Institutioner, Avhandlinger 103.
Ba M.B., Frouin R. and Nicholson S.E., 1995: Satellite derived inter-annual variability of West African Rainfall during 1983–88. Journal of Applied Meteorology 34 : 411–431.
Beran M.A. and Rodier J.A., 1985: Hydrological aspects of drought. Studies and Reports in Hydrology 39. UNESCO-WMO.
Binns T., 1990: Is desertification a myth? Geography 75 : 106–113.
Bley J., Van der Ploeg R.R., Sivakumar M.V.K. and Allison B.E., 1991: A risk probability map for millet production in southwest niger. In: Sivakumar M.V.K., Wallace J.S., Renard C. and Giroux C. (eds) 19 Soil Water Balance in the Sudano-Sahelian Zone . IAHS Publication 199, Wallingford, pp. 571–581.
Bruins H.J. and Berliner, P.R., 1998: Biocimatic aridity, climatic variability, drought and desertification: Definitions and management options. In: Bruins H.J. and Lithwick (eds), The Arid Frontier . Kluwer Academic Publishers, Dordrecht, pp. 97–116.
Chappell A. and Agnew C.T, 1999: Geostatistical analysis and simulation of West African rainfall. Proceedings International Geographical Union Commission on Land Degradation and Desertification 6th Meeting . Perth, University of Western Australia, 1999, Sept. 20–28.
Clausen B., 1995: Discharge data collection and analysis strategies inlow flow studies. Nordic Hydrology 26 : 191–204.
Cocheme J. and Franquin P., 1967: An agroclimatology survey of a semiarid area in Africa south of the Sahara. Technical Note 86, WMO, Geneva.
Copans J., 1983: The Sahelian drought. In: Hewitt K. (ed.), Interpretations of Calamity . Allen and Unwin, London, pp. 83–97.
D'Amato N. and Lebel T., 1998: On the characteristics of the rainfall events in the Sahel with a view to the analysis of climatic variability. International Journal of Climatology 18 : 955–974.
Davy E.G., Mattei F and Solomon S.I., 1976: An evaluation of the climate and water resources for development of agriculture in the Sudan-Sahelizn zone of West Africa WMO Special Environmental Report No. 9. WMO, Geneva.
Dracup J., Lee K. and Paulson E., 1980: On the definition of droughts. Water Resources Research 16 (2): 297–302.
Flohn H., 1987: Rainfall teleconnections in Northern and North Eastern Africa. Theoretical and Applied Climatology 38 : 191–197.
Franke F. and Chasin B., 1980: Seeds of Famine . Allanheld, Osman and Co., New Jersey.
Garcia R.V., 1981: Drought and Man . Volume 1. Nature Pleads Not Guilty . Pergamon, Oxford.
Glantz M. (ed.), 1987: Drought and Hunger in Africa . Cambridge University Press, Cambridge.
Glantz M. (ed.), 1994: Drought Follows the Plow . Cambridge University Press, Cambridge.
Gordon A.H., 1993: The random nature of drought: mathematical and physical causes. International Journal of Climatology 13 : 497–507.
Gustard A., Bullock A. and Dixon J.M., 1992: Low flow estimation in the U.K. Institute of Hydrology Report 108, Wallingford.
Hare F.K., 1984: Climate, drought and desertification. Nature and Resources ( UNECSO ) 20 (1): 2–8.
Hulme M., 1992: Rainfall changes in Africa. International Journal of Climatology 12 : 685–699.
Hulme M., 1996: Recent climatic change in the world's drylands. Geophysical Research Letters 23 (1): 61–64.
Hulme M. and Kelly M., 1993: Desertification and climate change. Environment 35 (6): 39–45.
International Union for Conservation of Nature 1989: Sahel Studies. IUCN, Nairobi.
Janicot S., Harzallah A., Fontaine B. and Moron V., 1998: West African monsoon dynamics and eastern equitorial atlantic and pacific SST anomalies (1970–88). Journal of Climate 11 : 1874–1882.
Jones P.D. and Hulme M., 1996: Calculating regional climatic time series for temperature and precipitation: methods and illustrations 16 : 361–377.
Kelly M. and Buchanan-Smith M., 1994: North Sudan in 1991: food crisis and the international relief response. Disasters 18 (1): 16–34.
Lamb H.H., 1974 The Earth's changing climate. Ecologist 4 : 10–15.
Le Houerou H.N., 1996: Climate change, drought and desertification. Journal of Arid Environments 34 : 133–185.
MacDonald L.H., 1986: Natural resources development in the Sahel: the role of the United Nations System. United Nations University, Japan.
Mainguet M, 1991: Desertification: Natural Background and Human Mismanagement . Springer-Verlag, Berlin.
Mainguet M., 1999: Aridity: Droughts and Human Development . Springer-Verlag, Berlin.
McKee T.B., Doesken N.J. and Kleist J., 1994: Drought monitoring with multiple time scales. Proceedings of Ninth Conference on Applied Climatology . American Meteorological Society, pp. 233–236.
Nicholson S.E. and Palao I.M., 1993: A re-evaluation of rainfall variability in the Sahel. International Journal Climatology 13 : 371–389.
Norse D., 1994: Multiple threats to regional food production:environment, economy, population? Food Policy 19 (2): 113–148.
Odingo R.S., 1992: Implementation of the plan of action to combat desertification (PACD) 1987–1991. Desertification Control Bulletin 21 : 6–14.
Olsson L., 1993: On the causes of famine — drought, desertification and market failure in the Sudan. Ambio 22 (6): 395–403.
Palmer W.C., 1965: Meteorological Drought US Weather Bureau Research Paper 45. Washington D.C.
Pritchard J.M., 1990: Africa . Longman, Harlow.
Raynaut C. (ed.), 1997: Societies and Nature in the Sahel . Routledge, London.
Sivakumar M.V.K., 1986: The climate of Niamey Resource Management Programme Progress Report 1. ICRISAT Sahelian Center BP 12404 Niamey.
Sivakumar M.V.K., 1989: Agroclimatic aspects of rainfed agriculture in the Sudano-Sahelina zone. In: Proceedings of Workshop on Soil, Crop and Water Management Systems for Rainfed Agriculture in the Sudano-Sahelian Zone , Niamey January 1987. ICRISAT Sahelian Center, Niamey. ICRISAT, Patancheru, AP 502 324 India, pp. 17–38.
Sivakumar M.V.K., and Wallace J.S., 1991: Soil water balance in the Sudano-Sahelian zone: need, relevance and objectives of the workshop. In: Sivakumar M.V.K., Wallace J.S., Renard C. and Giroux C. Soil water balance in the Sudano-Sahelian zone. IAHS Publication 199, Wallingford, pp. 3–10.
Tannehill I.R., 1947: Drought its Causes and Effects . Princeton University Press.
Thomas D.G., 1993: Sandstorm in a teacup: Understanding desertification. Geographical Journal 159 (3): 318–331.
Thomas D.G. and Middleton N., 1994: Desertification: Exploding the Myth . J. Wiley, Chichester.
Tickell C., 1986: Drought in Africa: impact and response. Overseas Development 102 : 10.
Tooze S., 1984: Sahel drought, call for joint action. Nature 307 : 497.
Toulmin C., 1988: Smiling in the Sahel. New Scientist 12 : 69.
UNEP 1992: World Atlas of Desertification , United Nations Environment Programme. Edward Arnold, London.
Warren A. and Agnew C.T., 1988: An assessment of desertification and land degradation in arid and semi arid areas. Paper 2, International Institute for Environment and Development, London.
Warren A., 1998: Environmental science and desertification. In: Bruins H.J. and Lithwick (eds), The Arid Frontier , Kluwer Academic Publishers, Dordrecht, pp. 117–127.
Wijkman A. and Timberlake L., 1985: Is the African drought an act of God or of Man? Ecologist 15 (1/2): 9–18.
Wilhite D.A. (ed.), 1993: Drought Assessment, Management and Planning: Theory and Case Studies . Kluwer Academic Publishers Boston, MA.
Winstanley D.W., 1973: Rainfall patterns and general atmospheric circulation. Nature 245 : 190–194.
Zheng X. and Eltahir E.A.B., 1998: The role of vegetation in the dynamics of west african monsoons. Journal of Climate 11 : 2078–2096.
Download references
Author information
Authors and affiliations.
School of Geography, Manchester University, Oxford Road, Manchester, M13 9PL, U.K., e-mail
Telford Institute of Environmental Systems, Department of Geography, University of Salford, Manchester, M5 4WT, U.K., e-mail
A. Chappell
You can also search for this author in PubMed Google Scholar
Rights and permissions
Reprints and permissions
About this article
Agnew, C., Chappell, A. Drought in the Sahel. GeoJournal 48 , 299–311 (1999). https://doi.org/10.1023/A:1007059403077
Download citation
Issue Date : August 1999
DOI : https://doi.org/10.1023/A:1007059403077
Share this article
Anyone you share the following link with will be able to read this content:
Sorry, a shareable link is not currently available for this article.
Provided by the Springer Nature SharedIt content-sharing initiative
- desertification
- desiccation
- drought classes
- meteorological drought
- Find a journal
- Publish with us
- Track your research
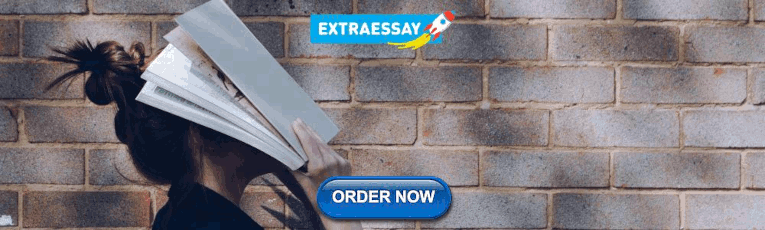
IMAGES
VIDEO
COMMENTS
Case study - the impact of drought in a developing country: the Sahel The Sahel is located directly south of the Sahara desert and stretches from the east to the west of Africa.
Sahel, where societies are organised simply and dry conditions are prolonged, the worst impacts of drought are felt. This Geofile examines the causes and consequences of drought and the way in which this hazard can be managed. Types of drought A variety of drought types can be recognised (see Figure 1). Four are outlined below: 1.
Understanding the Sahel drought of the late 20th century and improving our future projections for the hydrological cycle in this region is a major challenge for climate dynamics and climate modeling. ... For a more recent influential paper making a strong case for the dominance of oceanic forcing ... A variety of modeling studies have pointed ...
This paper is a critical synthesis of the causes, effects and challenges of the Sahelian droughts. The results show that the four main causes of the Sahelian droughts are as follows: sea surface temperature changes, vegetation and land degradation, dust feedbacks and human-induced climate change. However, human-induced climate change is seen as the major drought-determining factor because it ...
Between the 1960s and 1980s severe drought plagued the Sahel, resulting in widespread famine and displacement. Although rainfall has subsequently increased in the region, it is not known whether ...
Since the late 1960s, the Sahel—a semiarid region in West Africa between the Sahara desert and the Guinea coast rainforest—has experienced a drought of unprecedented severity in recorded history. The drought has had a devastating impact on this ecologically vulnerable region and was a major impetus in the establishment of the United Nations Convention on Combating Desertification and ...
The Sahel has been the subject of extensive study as a result of an extended period of desiccation commencing in the late 1960s and continuing into the 1990s (Hulme, 1996, 2001).
As climate model uncertainties remain very large for future rainfall in the Sahel, a multi-centennial perspective is required to assess the situation of current Sahel climate in the context of global warming. We present here the first record of hydroclimatic variability over the past 1600 years in Senegal, obtained from stable oxygen isotope analyses (δ18O) in archaeological shell middens ...
The association between warming of the tropical oceans and Sahel drought opens up for consideration of drought and desertification in the context of global climate change (see also Kerr, 2003, ... Further case studies of tropical Atlantic surface atmospheric and oceanic patterns associated with sub-Saharan drought. J. Climate, 5 (1992), pp. 476 ...
Abstract. This paper synthesizes a set of national case studies conducted in the Sahelian countries during 2019-2020 as a collaboration between national universities and research institutes, and the Center for Development Research (ZEF), University of Bonn, with contributions from the Agrhymet Regional Centre, Permanent Interstate Committee for Drought Control in the Sahel (CILSS).
One of the first environmental crises to attract interest in development initiatives and aid was the great drought of the 1970s in the Sahel. This study investigates the extent of damage caused by natural disasters from one of the most widely used databases—EM-DAT—with a sample size of 16 Sahelian countries over the period 1960-2020. These countries have been divided into three regions ...
Early concern focused on the influence of land-use practices 2, but later observational 3 and modelling 4,5,6 studies related decadal-scale Sahel drought with sea surface temperatures ...
23 January 2022 Humanitarian Aid. Millions of hectares of farmland are lost to the desert each year in Africa's Sahel region, but the UN Food and Agriculture Organization (FAO) is showing that traditional knowledge, combined with the latest technology, can turn arid ground back into fertile soil. Those trying to grow crops in the Sahel region ...
A composite drought index (CDI) was developed for seasonal drought monitoring at 1 km 2 resolution over the West African Sahel (WAS). The CDI was derived from remote sensing data, mainly, the Climate Hazards Group InfraRed Precipitation with Stations (CHIRPS), normalized difference vegetation index (NDVI) and land surface temperature (LST) from the Terra/MODIS satellite.
The Sahel region of Africa has long experienced a series of historic droughts, dating back to at least the 17th century. The Sahel region is a climate zone sandwiched between the Sudanian Savanna to the south and the Sahara desert to the north, across West and Central Africa. While the frequency of drought in the region is thought to have ...
The Sahel is a major global agroecological region and its success in adaptation will influence the achievement of the global Millennium Development Goals. From simple typologies of adaptation strategies, our understanding of adaptive capacity has evolved over time (with accumulating observations) into a contextual model which places drought ...
Abstract The Sahel experienced a severe drought during the 1970s and 1980s after wet periods in the 1950s and 1960s. Although rainfall partially recovered since the 1990s, the drought had devastating impacts on society. Most studies agree that this dry period resulted primarily from remote effects of sea surface temperature (SST) anomalies amplified by local land surface-atmosphere interactions.
Scientists say that the current drought in the Sahel began as far back the 1960s. Between the vast Sahara Desert and the dense foliage of the African Rainforest is a band of semi-arid grassland ...
In the South Sahel, precipitation was the most important driver of increasing stability, followed by the tree canopy (Figure 5a). ... This study assessed the drought resistance and resilience using remotely sensed vegetation data sets, clarified that the tradeoff between resistance and resilience existed globally and found a temporal decline in ...
The 1983 drought in the West Sahel: a case study. Ju¨rgen Bader•Mojib Latif. Received: 3 June 2009/Accepted: 27 October 2009/Published online: 12 November 2009 Springer-Verlag 2009. Abstract Some drought years over sub-Saharan west Africa (1972, 1977, 1984) have been previously related to a cross-equatorial Atlantic gradient pattern with ...
The northern Sahel region is experiencing a 30-40% annual departure from normal rainfall. 25-30% below that, and 20-25% in the southern Sahel. Human factors act like a feedback loop. Humans enhance the impacts of drought by the over-abstraction of surface water from rivers and ponds, and of groundwater from aquifers. Key human factors ...
The drought is worsening water shortages, potentially affecting 2.6 million people. In view of the worsening situation, the Emergency Relief Coordinator, Martin Griffiths, released $13.5 million last month from the Central Emergency Response Fund to address the El Niño-induced drought in Southern Africa.
induced global warming, a causal mechanism linking human activity and drought in the Sahel cannot be proven, and models of future climate change suggest that such warming ... The Sahel has been the subject of extensive study as a result of an extended period of desiccation commencing in the late 1960s and continuing into the 1990s (Hulme, 1996,
The Central Sahel is the most affected by violence, with many civilians killed in 2022 alone. 69 The Central Sahel was thrown into further turmoil in 2021 after military coups in Burkina Faso and Mali, which resulted in their suspensions from the Economic Community of West African States (ECOWAS) and the African Union. 70 In 2022, there were ...
Some drought years over sub-Saharan west Africa (1972, 1977, 1984) have been previously related to a cross-equatorial Atlantic gradient pattern with anomalously warm sea surface temperatures (SSTs) south of 10°N and anomalously cold SSTs north of 10°N. This SST dipole-like pattern was not characteristic of 1983, the third driest summer of the twentieth century in the Sahel. This study ...
Based on results from small scale pilot studies, techno economic analysis indicates that the Linde/BASF process can provide a significant reduction in capital costs compared to the NETL base case for a supercritical pulverized coal plant with carbon capture." Multiple other FEED studies are either completed or under development, putting those ...
The Sahel region of West Africa is well known as a region of environmental degradation. The reported incidence of desertification has been challenged but persistent and widespread drought is still widely accepted. Drought, defined solely as a function of rainfall, is believed to have commenced in the early 1970s and continued through to the present. It is usually defined as a meteorological ...