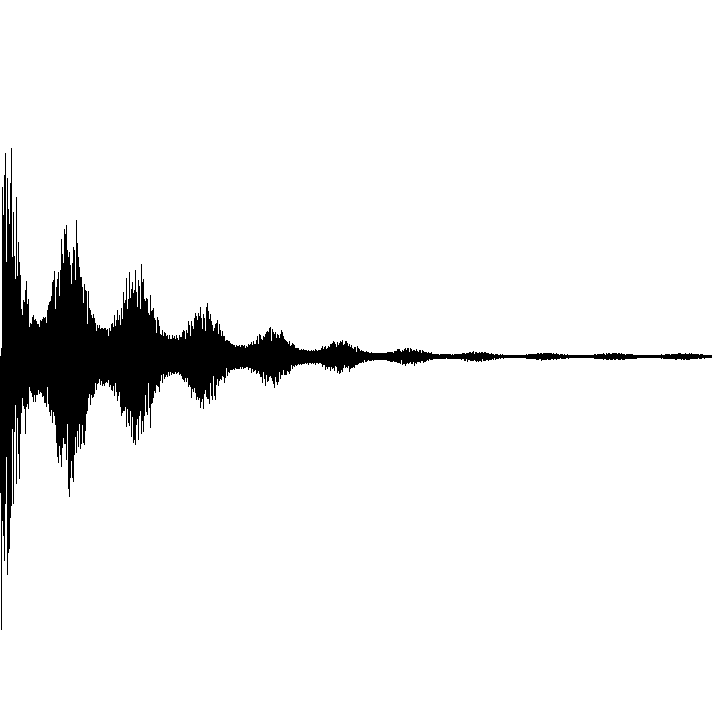
When you look at an NMR spectrum, do you see only a bunch of disordered lines or peaks? Then you have come to the right place. This site was established to provide people interested in NMR with a library of NMR spectroscopy problems. Interpretation of spectra is a skill that requires pattern recognition and/or practice to master the chaos. This site provides one dimensional spectra of different nuclei, COSY, HSQC, HMBC and some less common spectra of various compounds for you to interpret, together with worked solutions. Hopefully, these problems will provide a useful resource to help you better understand NMR spectral interpretation.
A series of about 50 problems is available in printable form. The print version will not be developed further. The PDF documents are still available (german only). Get them here:
The step-by-step approach works nearly perfectly on the desktop and most tablets. On cellular phones, PowerPoint documents are sometimes displayed incorrectly. There is a workaround, but it is unfortunately much too complicated to be practical. As an alternative, all documents can be saved locally. Feel free to follow the download links. A free PowerPoint viewer is available in both the iOS and Android AppStores. As a bonus, there would be no need for a permanent internet connection. Of course, this offline method also works with desktop computers.
The author is always grateful for criticism, suggestions, references to errors and information about the design via email:
Home | Courses | Preview | Institution | --> Certification | --> Parents | Clients | Support | About Blog -->
Chemistry : Physics : Biology : Math : SAT : ACT : AP : CLEP : MCAT : DAT : OAT : PCAT : Nursing : USMLE
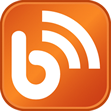
Trustlink is a Better Business Bureau Program. Rapid Learning Center is a fivr-star business. External TrustLink Reviews
Send a gift of education to someone you care. Give the learning edge with our rapid learning courses.
- RL-700-GP Premium
- RL-700-GS Standard
- RL-700-GL Lite
- Organic Chemistry
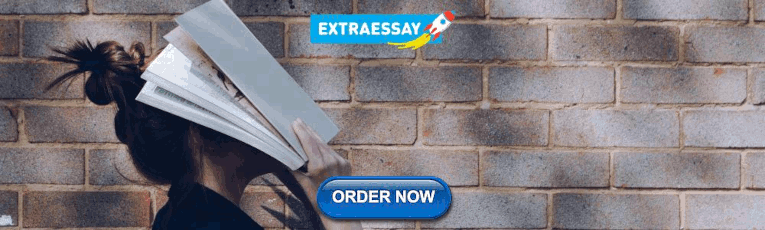
My Channels
- General Chemistry
- Analytical Chemistry
- GOB Chemistry
- Biochemistry
- Intro to Chemistry
- General Biology
- Microbiology
- Anatomy & Physiology
- Cell Biology
- College Algebra
- Trigonometry
- Precalculus
- Microeconomics
- Macroeconomics
- Financial Accounting
Social Sciences
Programming.
- Introduction to Python
- Microsoft Power BI
- Data Analysis - Excel
- Introduction to Blockchain
- HTML, CSS & Layout
- Introduction to JavaScript
- R Programming
Product & Marketing
- Agile & Product Management
- Digital Marketing
- Project Management
- AI in Marketing
Improve your experience by picking them
- Summary ( 0 )
- Intro to Organic Chemistry ( 0 )
- Atomic Structure ( 0 )
- Wave Function ( 0 )
- Molecular Orbitals ( 0 )
- Sigma and Pi Bonds ( 0 )
- Octet Rule ( 0 )
- Bonding Preferences ( 0 )
- Formal Charges ( 0 )
- Skeletal Structure ( 0 )
- Lewis Structure ( 0 )
- Condensed Structural Formula ( 0 )
- Degrees of Unsaturation ( 0 )
- Constitutional Isomers ( 0 )
- Resonance Structures ( 0 )
- Hybridization ( 0 )
- Molecular Geometry ( 0 )
- Electronegativity ( 0 )
- Intermolecular Forces ( 0 )
- How To Determine Solubility ( 0 )
- Functional Groups ( 0 )
- Organic Chemistry Reactions ( 0 )
- Reaction Mechanism ( 0 )
- Acids and Bases ( 0 )
- Equilibrium Constant ( 0 )
- Acid Base Equilibrium ( 0 )
- Ranking Acidity ( 0 )
- IUPAC Naming ( 0 )
- Alkyl Groups ( 0 )
- Naming Cycloalkanes ( 0 )
- Naming Bicyclic Compounds ( 0 )
- Naming Alkyl Halides ( 0 )
- Naming Alkenes ( 0 )
- Naming Alcohols ( 0 )
- Naming Amines ( 0 )
- Cis vs Trans ( 0 )
- Conformational Isomers ( 0 )
- Newman Projections ( 0 )
- Drawing Newman Projections ( 0 )
- Barrier To Rotation ( 0 )
- Ring Strain ( 0 )
- Axial vs Equatorial ( 0 )
- Cis vs Trans Conformations ( 0 )
- Equatorial Preference ( 0 )
- Chair Flip ( 0 )
- Calculating Energy Difference Between Chair Conformations ( 0 )
- A-Values ( 0 )
- Decalin ( 0 )
- Constitutional Isomers vs. Stereoisomers ( 0 )
- Chirality ( 0 )
- Test 1:Plane of Symmetry ( 0 )
- Test 2:Stereocenter Test ( 0 )
- R and S Configuration ( 0 )
- Enantiomers vs. Diastereomers ( 0 )
- Atropisomers ( 0 )
- Meso Compound ( 0 )
- Test 3:Disubstituted Cycloalkanes ( 0 )
- What is the Relationship Between Isomers? ( 0 )
- Fischer Projection ( 0 )
- R and S of Fischer Projections ( 0 )
- Optical Activity ( 0 )
- Enantiomeric Excess ( 0 )
- Calculations with Enantiomeric Percentages ( 0 )
- Non-Carbon Chiral Centers ( 0 )
- Energy Diagram ( 0 )
- Gibbs Free Energy ( 0 )
- Enthalpy ( 0 )
- Entropy ( 0 )
- Hammond Postulate ( 0 )
- Carbocation Stability ( 0 )
- Carbocation Intermediate Rearrangements ( 0 )
- Nucleophilic Substitution ( 0 )
- Good Leaving Groups ( 0 )
- SN2 Reaction ( 0 )
- SN1 Reaction ( 0 )
- Substitution Comparison ( 0 )
- E2 Mechanism ( 0 )
- Beta Hydrogen ( 0 )
- E2 - Anti-Coplanar Requirement ( 0 )
- E2 - Cumulative Practice ( 0 )
- E1 Reaction ( 0 )
- Solvents ( 0 )
- Leaving Groups ( 0 )
- Nucleophiles and Basicity ( 0 )
- SN1 SN2 E1 E2 Chart (Big Daddy Flowchart) ( 0 )
- Cumulative Substitution/Elimination ( 0 )
- Alkene Stability ( 0 )
- Zaitsev Rule ( 0 )
- Dehydrohalogenation ( 0 )
- Double Elimination ( 0 )
- Acetylide ( 0 )
- Hydrogenation of Alkynes ( 0 )
- Dehydration Reaction ( 0 )
- POCl3 Dehydration ( 0 )
- Alkynide Synthesis ( 0 )
- Addition Reaction ( 0 )
- Markovnikov ( 0 )
- Hydrohalogenation ( 0 )
- Acid-Catalyzed Hydration ( 0 )
- Oxymercuration ( 0 )
- Hydroboration ( 0 )
- Hydrogenation ( 0 )
- Halogenation ( 0 )
- Halohydrin ( 0 )
- Carbene ( 0 )
- Epoxidation ( 0 )
- Epoxide Reactions ( 0 )
- Dihydroxylation ( 0 )
- Ozonolysis ( 0 )
- Ozonolysis Full Mechanism ( 0 )
- Oxidative Cleavage ( 0 )
- Alkyne Oxidative Cleavage ( 0 )
- Alkyne Hydrohalogenation ( 0 )
- Alkyne Halogenation ( 0 )
- Alkyne Hydration ( 0 )
- Alkyne Hydroboration ( 0 )
- Radical Reaction ( 0 )
- Radical Stability ( 0 )
- Free Radical Halogenation ( 0 )
- Radical Selectivity ( 0 )
- Calculating Radical Yields ( 0 )
- Anti Markovnikov Addition of Br ( 0 )
- Free Radical Polymerization ( 0 )
- Allylic Bromination ( 0 )
- Radical Synthesis ( 0 )
- Alcohol Nomenclature ( 0 )
- Naming Ethers ( 0 )
- Naming Epoxides ( 0 )
- Naming Thiols ( 0 )
- Alcohol Synthesis ( 0 )
- Leaving Group Conversions - Using HX ( 0 )
- Leaving Group Conversions - SOCl2 and PBr3 ( 0 )
- Leaving Group Conversions - Sulfonyl Chlorides ( 0 )
- Leaving Group Conversions Summary ( 0 )
- Williamson Ether Synthesis ( 0 )
- Making Ethers - Alkoxymercuration ( 0 )
- Making Ethers - Alcohol Condensation ( 0 )
- Making Ethers - Acid-Catalyzed Alkoxylation ( 0 )
- Making Ethers - Cumulative Practice ( 0 )
- Ether Cleavage ( 0 )
- Alcohol Protecting Groups ( 0 )
- t-Butyl Ether Protecting Groups ( 0 )
- Silyl Ether Protecting Groups ( 0 )
- Sharpless Epoxidation ( 0 )
- Thiol Reactions ( 0 )
- Sulfide Oxidation ( 0 )
- Oxidizing and Reducing Agents ( 0 )
- Oxidizing Agent ( 0 )
- Reducing Agent ( 0 )
- Nucleophilic Addition ( 0 )
- Preparation of Organometallics ( 0 )
- Grignard Reaction ( 0 )
- Protecting Alcohols from Organometallics ( 0 )
- Organometallic Cumulative Practice ( 0 )
- Synthetic Cheatsheet ( 0 )
- Moving Functionality ( 0 )
- Alkynide Alkylation ( 0 )
- Alkane Halogenation ( 0 )
- Retrosynthesis ( 0 )
- Purpose of Analytical Techniques ( 0 )
- Infrared Spectroscopy ( 0 )
- Infrared Spectroscopy Table ( 0 )
- IR Spect:Drawing Spectra ( 0 )
- IR Spect:Extra Practice ( 0 )
- NMR Spectroscopy ( 0 )
- 1H NMR:Number of Signals ( 0 )
- 1H NMR:Q-Test ( 0 )
- 1H NMR:E/Z Diastereoisomerism ( 0 )
- H NMR Table ( 0 )
- 1H NMR:Spin-Splitting (N + 1) Rule ( 0 )
- 1H NMR:Spin-Splitting Simple Tree Diagrams ( 0 )
- 1H NMR:Spin-Splitting Complex Tree Diagrams ( 0 )
- 1H NMR:Spin-Splitting Patterns ( 0 )
- NMR Integration ( 0 )
- NMR Practice ( 0 )
- Carbon NMR ( 0 )
- Structure Determination without Mass Spect ( 0 )
- Mass Spectrometry ( 0 )
- Mass Spect:Fragmentation ( 0 )
- Mass Spect:Isotopes ( 0 )
- Conjugation Chemistry ( 0 )
- Stability of Conjugated Intermediates ( 0 )
- Allylic Halogenation ( 0 )
- Conjugated Hydrohalogenation (1,2 vs 1,4 addition) ( 0 )
- Diels-Alder Reaction ( 0 )
- Diels-Alder Forming Bridged Products ( 0 )
- Diels-Alder Retrosynthesis ( 0 )
- Molecular Orbital Theory ( 0 )
- Drawing Atomic Orbitals ( 0 )
- Drawing Molecular Orbitals ( 0 )
- HOMO LUMO ( 0 )
- Orbital Diagram:3-atoms- Allylic Ions ( 0 )
- Orbital Diagram:4-atoms- 1,3-butadiene ( 0 )
- Orbital Diagram:5-atoms- Allylic Ions ( 0 )
- Orbital Diagram:6-atoms- 1,3,5-hexatriene ( 0 )
- Orbital Diagram:Excited States ( 0 )
- Pericyclic Reaction ( 0 )
- Thermal Cycloaddition Reactions ( 0 )
- Photochemical Cycloaddition Reactions ( 0 )
- Thermal Electrocyclic Reactions ( 0 )
- Photochemical Electrocyclic Reactions ( 0 )
- Cumulative Electrocyclic Problems ( 0 )
- Sigmatropic Rearrangement ( 0 )
- Cope Rearrangement ( 0 )
- Claisen Rearrangement ( 0 )
- Aromaticity ( 0 )
- Huckel's Rule ( 0 )
- Pi Electrons ( 0 )
- Aromatic Hydrocarbons ( 0 )
- Annulene ( 0 )
- Aromatic Heterocycles ( 0 )
- Frost Circle ( 0 )
- Naming Benzene Rings ( 0 )
- Acidity of Aromatic Hydrocarbons ( 0 )
- Basicity of Aromatic Heterocycles ( 0 )
- Ionization of Aromatics ( 0 )
- Electrophilic Aromatic Substitution ( 0 )
- Benzene Reactions ( 0 )
- EAS:Halogenation Mechanism ( 0 )
- EAS:Nitration Mechanism ( 0 )
- EAS:Friedel-Crafts Alkylation Mechanism ( 0 )
- EAS:Friedel-Crafts Acylation Mechanism ( 0 )
- EAS:Any Carbocation Mechanism ( 0 )
- Electron Withdrawing Groups ( 0 )
- EAS:Ortho vs. Para Positions ( 0 )
- Acylation of Aniline ( 0 )
- Limitations of Friedel-Crafts Alkyation ( 0 )
- Advantages of Friedel-Crafts Acylation ( 0 )
- Blocking Groups - Sulfonic Acid ( 0 )
- EAS:Synergistic and Competitive Groups ( 0 )
- Side-Chain Halogenation ( 0 )
- Side-Chain Oxidation ( 0 )
- Birch Reduction ( 0 )
- EAS:Sequence Groups ( 0 )
- EAS:Retrosynthesis ( 0 )
- Diazo Replacement Reactions ( 0 )
- Diazo Sequence Groups ( 0 )
- Diazo Retrosynthesis ( 0 )
- Nucleophilic Aromatic Substitution ( 0 )
- Benzyne ( 0 )
- Naming Aldehydes ( 0 )
- Naming Ketones ( 0 )
- Oxidation of Alcohols ( 0 )
- DIBAL ( 0 )
- Cyanohydrin ( 0 )
- Organometallics on Ketones ( 0 )
- Overview of Nucleophilic Addition of Solvents ( 0 )
- Hydrates ( 0 )
- Hemiacetal ( 0 )
- Acetal ( 0 )
- Acetal Protecting Group ( 0 )
- Thioacetal ( 0 )
- Imine vs Enamine ( 0 )
- Addition of Amine Derivatives ( 0 )
- Wolff Kishner Reduction ( 0 )
- Baeyer-Villiger Oxidation ( 0 )
- Acid Chloride to Ketone ( 0 )
- Nitrile to Ketone ( 0 )
- Wittig Reaction ( 0 )
- Ketone and Aldehyde Synthesis Reactions ( 0 )
- Carboxylic Acid Derivatives ( 0 )
- Naming Carboxylic Acids ( 0 )
- Diacid Nomenclature ( 0 )
- Naming Esters ( 0 )
- Naming Nitriles ( 0 )
- Acid Chloride Nomenclature ( 0 )
- Naming Anhydrides ( 0 )
- Naming Amides ( 0 )
- Nucleophilic Acyl Substitution ( 0 )
- Carboxylic Acid to Acid Chloride ( 0 )
- Fischer Esterification ( 0 )
- Acid-Catalyzed Ester Hydrolysis ( 0 )
- Saponification ( 0 )
- Transesterification ( 0 )
- Lactones, Lactams and Cyclization Reactions ( 0 )
- Carboxylation ( 0 )
- Decarboxylation Mechanism ( 0 )
- Tautomerization ( 0 )
- Tautomers of Dicarbonyl Compounds ( 0 )
- Enolate ( 0 )
- Acid-Catalyzed Alpha-Halogentation ( 0 )
- Base-Catalyzed Alpha-Halogentation ( 0 )
- Haloform Reaction ( 0 )
- Hell-Volhard-Zelinski Reaction ( 0 )
- Overview of Alpha-Alkylations and Acylations ( 0 )
- Enolate Alkylation and Acylation ( 0 )
- Enamine Alkylation and Acylation ( 0 )
- Beta-Dicarbonyl Synthesis Pathway ( 0 )
- Acetoacetic Ester Synthesis ( 0 )
- Malonic Ester Synthesis ( 0 )
- Condensation Reactions ( 0 )
- Aldol Condensation ( 0 )
- Directed Condensations ( 0 )
- Crossed Aldol Condensation ( 0 )
- Claisen-Schmidt Condensation ( 0 )
- Claisen Condensation ( 0 )
- Intramolecular Aldol Condensation ( 0 )
- Conjugate Addition ( 0 )
- Michael Addition ( 0 )
- Robinson Annulation ( 0 )
- Amine Alkylation ( 0 )
- Gabriel Synthesis ( 0 )
- Amines by Reduction ( 0 )
- Nitrogenous Nucleophiles ( 0 )
- Reductive Amination ( 0 )
- Curtius Rearrangement ( 0 )
- Hofmann Rearrangement ( 0 )
- Hofmann Elimination ( 0 )
- Cope Elimination ( 0 )
- Monosaccharide ( 0 )
- Monosaccharides - D and L Isomerism ( 0 )
- Monosaccharides - Drawing Fischer Projections ( 0 )
- Monosaccharides - Common Structures ( 0 )
- Monosaccharides - Forming Cyclic Hemiacetals ( 0 )
- Monosaccharides - Cyclization ( 0 )
- Monosaccharides - Haworth Projections ( 0 )
- Mutarotation ( 0 )
- Epimerization ( 0 )
- Monosaccharides - Aldose-Ketose Rearrangement ( 0 )
- Monosaccharides - Alkylation ( 0 )
- Monosaccharides - Acylation ( 0 )
- Glycoside ( 0 )
- Monosaccharides - N-Glycosides ( 0 )
- Monosaccharides - Reduction (Alditols) ( 0 )
- Monosaccharides - Weak Oxidation (Aldonic Acid) ( 0 )
- Reducing Sugars ( 0 )
- Monosaccharides - Strong Oxidation (Aldaric Acid) ( 0 )
- Monosaccharides - Oxidative Cleavage ( 0 )
- Monosaccharides - Osazones ( 0 )
- Monosaccharides - Kiliani-Fischer ( 0 )
- Monosaccharides - Wohl Degradation ( 0 )
- Monosaccharides - Ruff Degradation ( 0 )
- Disaccharide ( 0 )
- Polysaccharide ( 0 )
- Phenol Acidity ( 0 )
- Proteins and Amino Acids ( 0 )
- L and D Amino Acids ( 0 )
- Polar Amino Acids ( 0 )
- Amino Acid Chart ( 0 )
- Acid-Base Properties of Amino Acids ( 0 )
- Isoelectric Point ( 0 )
- Electron Configuration of Elements ( 0 )
- Coordination Complexes ( 0 )
- Ligands ( 0 )
- Electron Counting ( 0 )
- The 18 and 16 Electron Rule ( 0 )
- Cross-Coupling General Reactions ( 0 )
- Heck Reaction ( 0 )
- Stille Reaction ( 0 )
- Suzuki Reaction ( 0 )
- Sonogashira Coupling Reaction ( 0 )
- Fukuyama Coupling Reaction ( 0 )
- Kumada Coupling Reaction ( 0 )
- Negishi Coupling Reaction ( 0 )
- Buchwald-Hartwig Amination Reaction ( 0 )
- Eglinton Reaction ( 0 )
NMR Practice Practice Problems
Draw the 1 H NMR spectrum of the following molecule making sure to show the integration of each peak, without thinking about the relative placement of the signals (i.e., the chemical shift) or the splitting patterns. Label each signal according to the set of equivalent hydrogens to which it belongs.
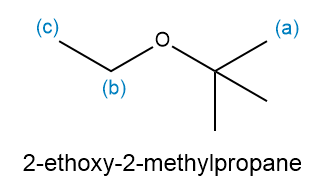
For the following compound, draw an estimated 1 H NMR spectrum, and show the multiplicity, integration, and chemical shift of each signal. Mark the signals according to the sets of equivalent hydrogens.
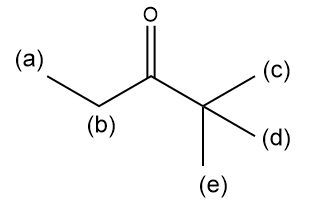
For the given compound, draw an estimated 1 H NMR spectrum, and show the multiplicity, integration, and chemical shift of each signal. Mark the signals according to the sets of equivalent hydrogens.
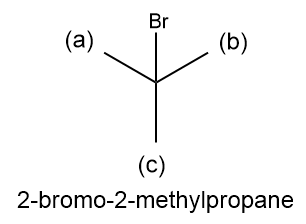
Draw the expected 1 H NMR spectrum for the following molecule.
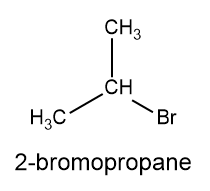
Match the peaks in the 1H NMR spectrum of the given molecule with their corresponding hydrogens.
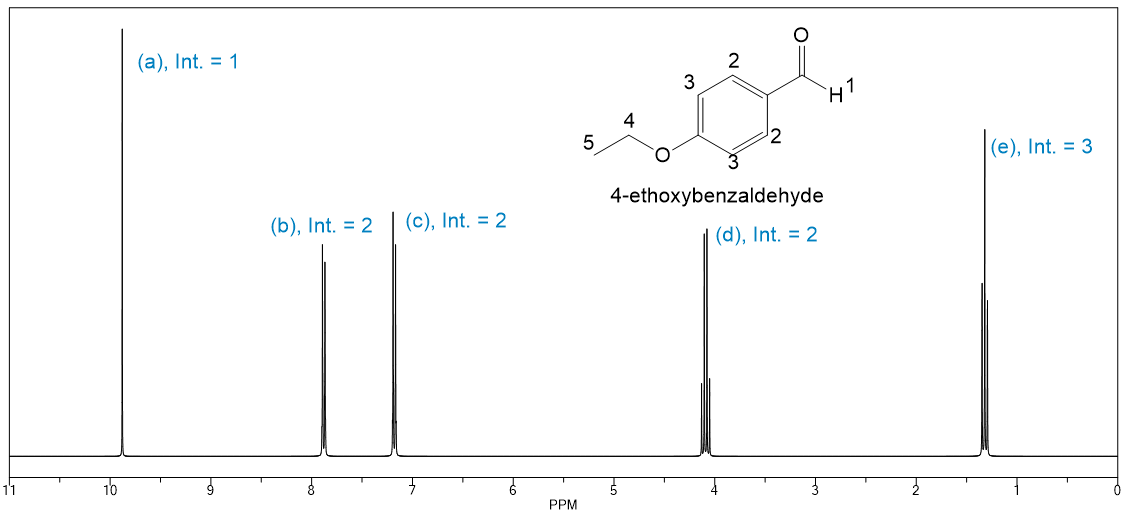
Produce a table of chemical shifts, integrations, and multiplicities of each 1 H NMR peak expected from the labeled protons of the following compound.
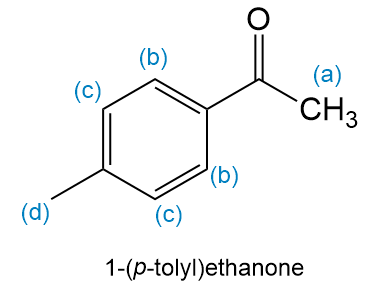
Draw the expected 1H NMR spectrum for the molecule shown below.
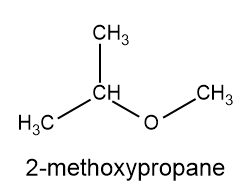
Draw the expected 1 H NMR spectrum for the following compound.
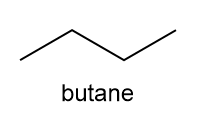
What is the structure of the compound that has the following 1 H NMR spectrum data and molecular formula of C 5 H 11 Cl?
1 H quintet at 3.38 ppm, 1 H octet at 1.91 ppm, 3 H doublet at 1.55 ppm, 6 H doublet at 0.88 ppm

Which compound produced the following 1 H NMR spectrum?
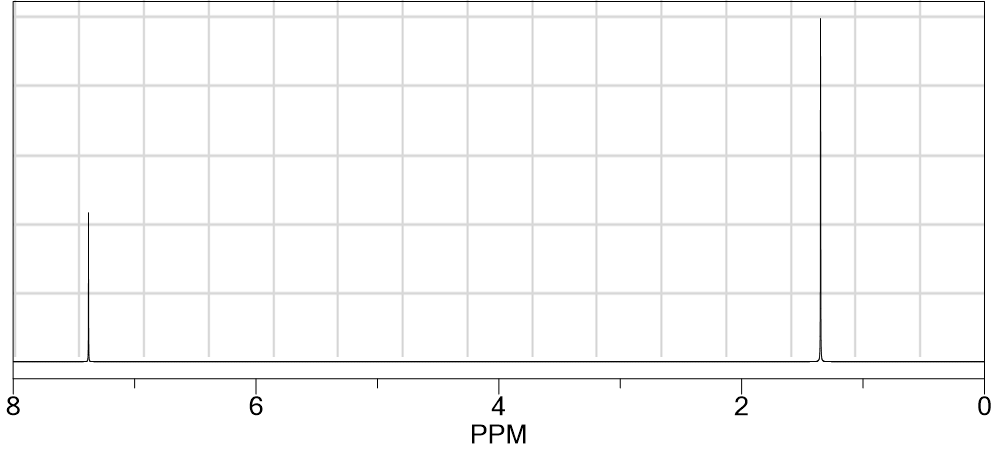
Describe the expected 1 H NMR spectrum and indicate the relative position of the signals for the given compound.
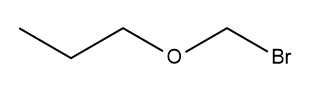
Describe the expected 1 H NMR spectrum and the relative position of the signals for the given compound.
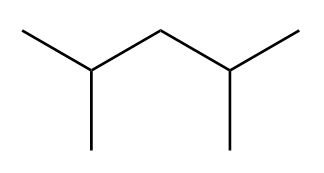
Determine the structure of the compound with the molecular formula C 14 H 22 O 2 , which produces the following spectrum. (Note: The integral ratios are given across the spectrum from left to right.)
A 1 H NMR spectrum containing two singlets with integral ratios of 2:9.
Differentiate two isomers (a primary alcohol and an ether) with the molecular formula C 3 H 8 O using 1 H NMR.
What are the chemically nonequivalent proton ratios in the compound if the integration curve steps from left to right across the spectrum are 61, 122, 61, and 366 mm? Which of the following compounds would exhibit this sequence of integrals in a 1 H NMR spectrum?
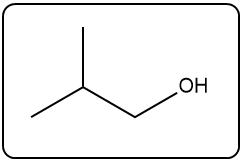
True or False. The 1 H NMR spectrum below corresponds to the given compound.
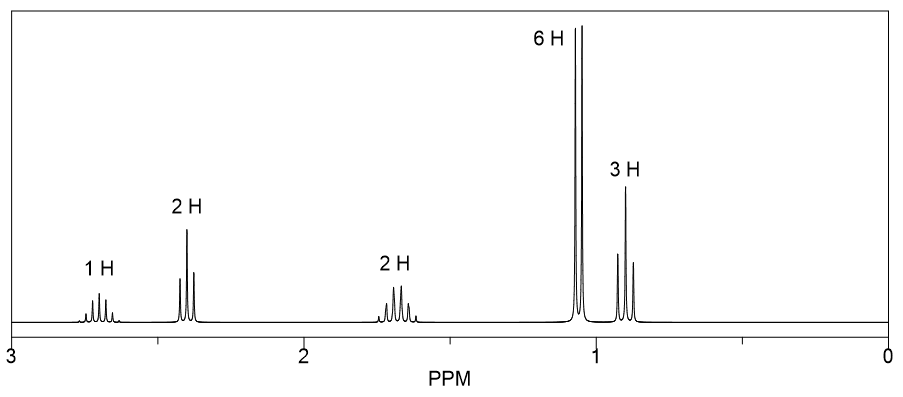
Which of the three isomers with the molecular formula C 6 H 11 Cl has the following 1 H NMR spectrum?
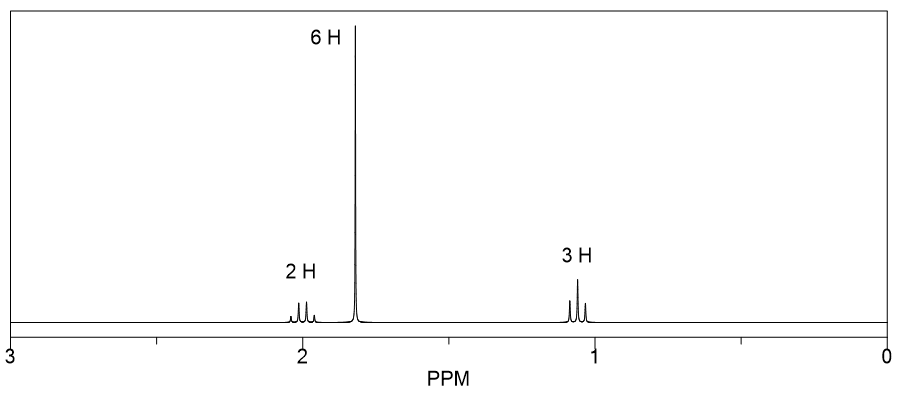
Which of the three isomers with the molecular formula C 5 H 8 Cl 2 has the following 1 H NMR spectrum?
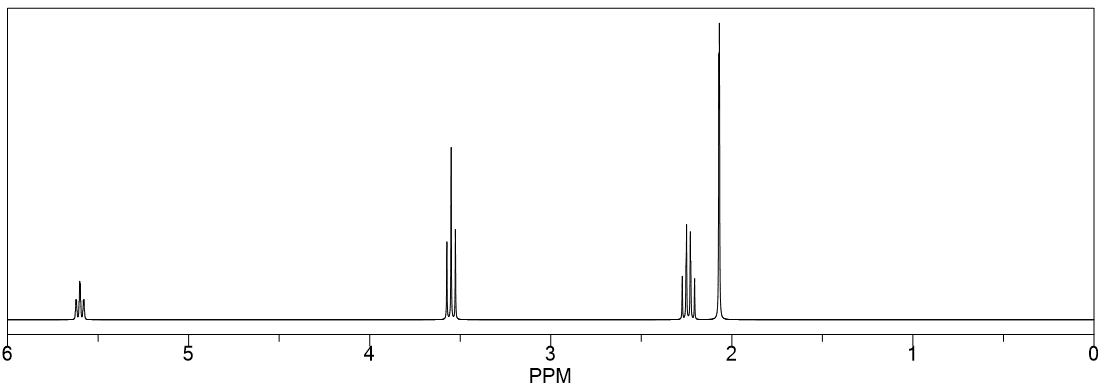
Which of the three isomers with the molecular formula C 5 H 10 Cl 2 has the following 1 H NMR spectrum?
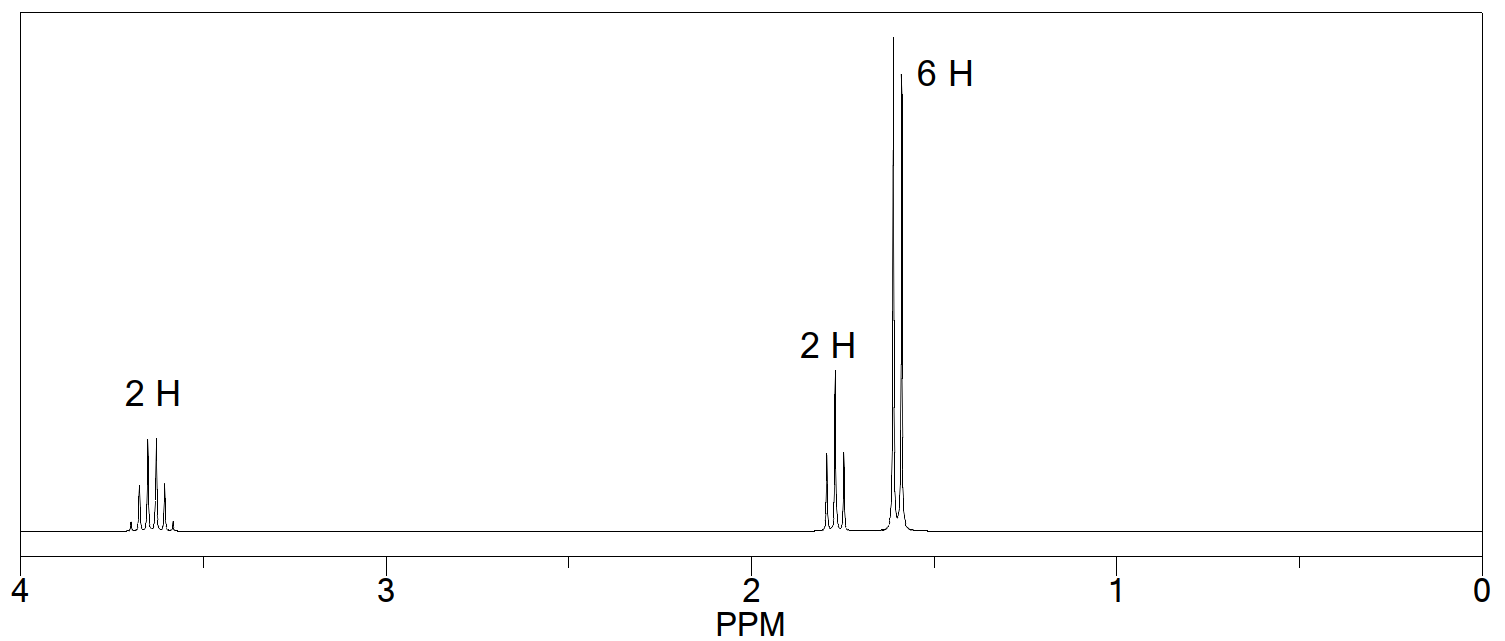
The 1 H NMR spectrum of the compound created by the reaction of an alkyl halide and an alkoxide ion is shown here. What are the structures of the alkyl halide and alkoxide ion?
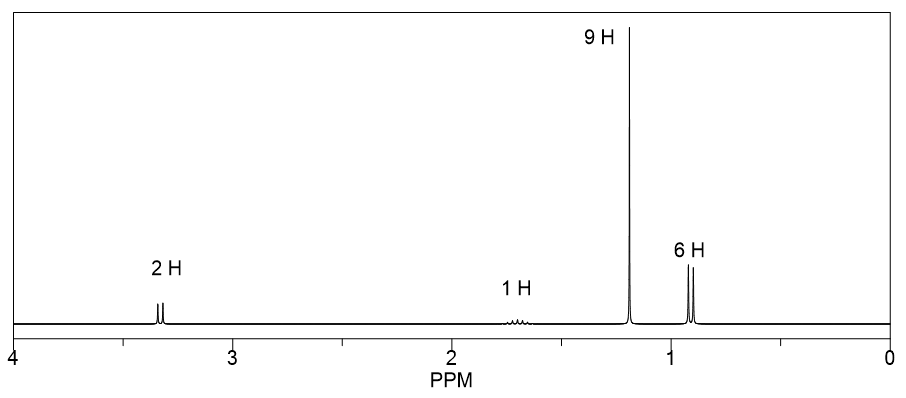
Which of the three isomers with the molecular formula C 6 H 12 O has the following 1 H NMR spectrum?
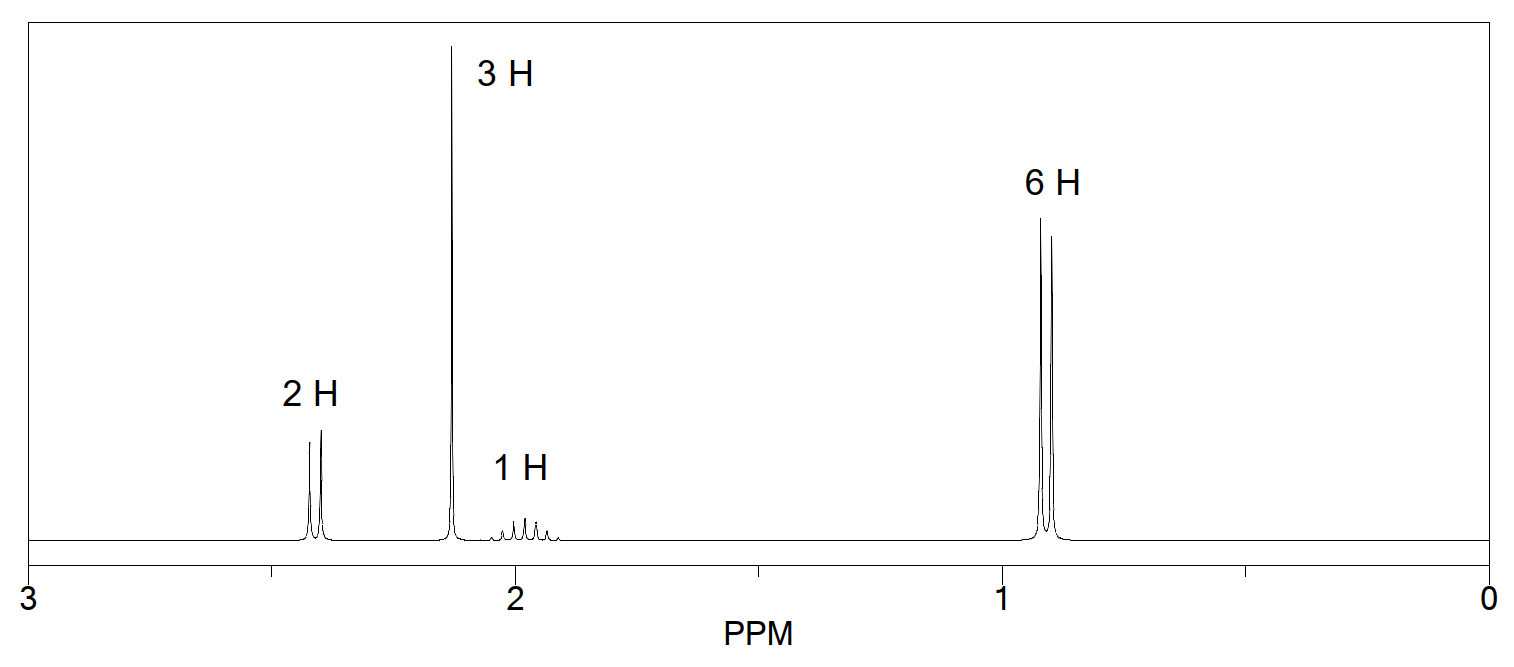
What is the structure of the compound with a molecular formula of C 4 H 8 O 2 that has the following 1 H NMR and IR spectra?
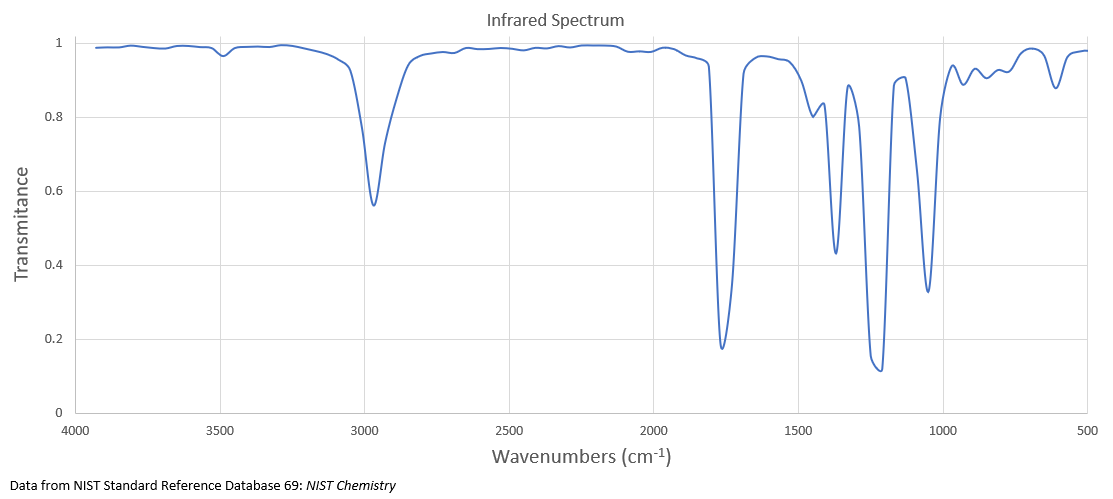
What is the structure of the compound with a molecular formula of C 4 H 8 O 2 that has the following 1 H NMR and IR spectra?
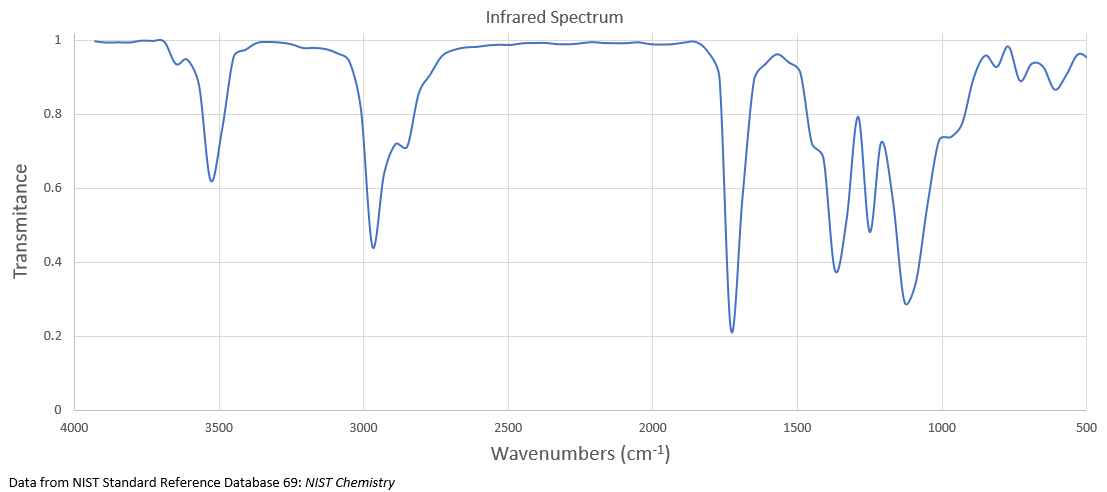
Assign the protons responsible for each of the signals in the 1 H NMR spectrum for 2,4-dimethyl-3-pentanol.
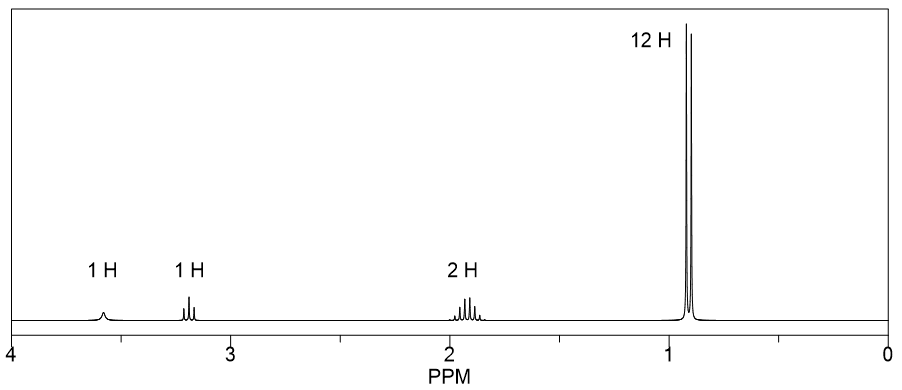
Describe the 1 H NMR spectrum for an anhydrous sample of 3-chloro-3-methylbutan-1-ol.
Describe the 1 H NMR spectrum for a sample of 3-chloro-3-methylbutan-1-ol with a trace amount of acid.
The 1 H NMR spectrum below is produced by a compound with the molecular formula C 5 H 10 O 2 . What is its structure?
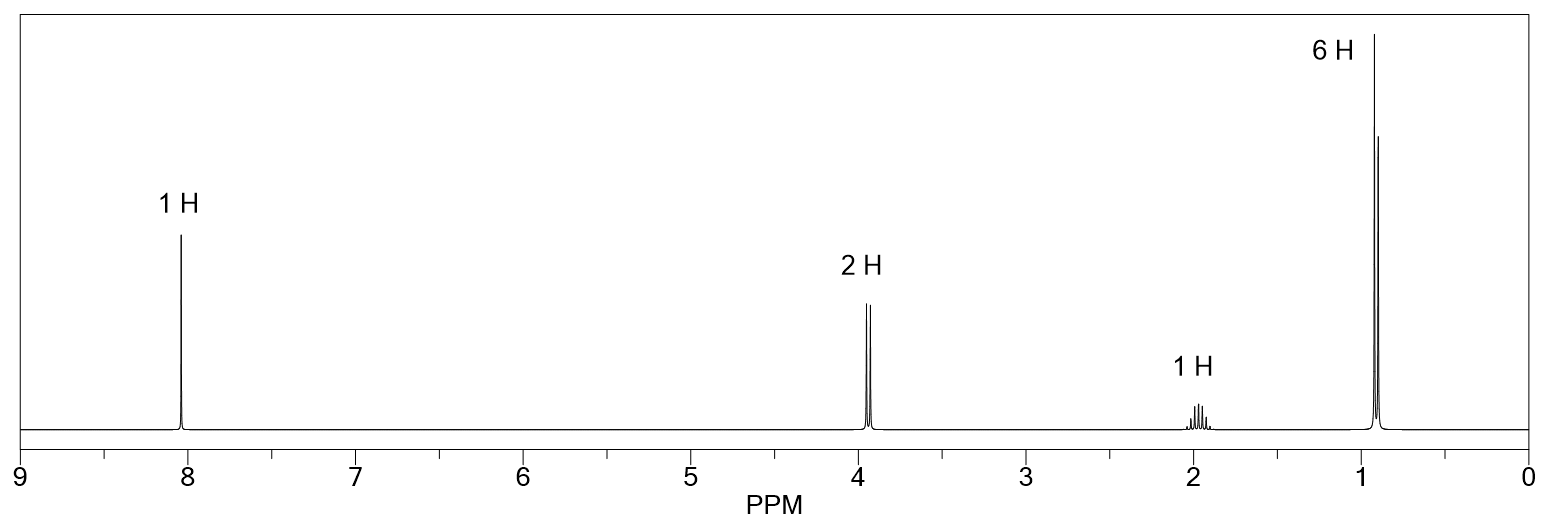
What is the structure of the compound with a molecular formula of C 9 H 18 O that has the following 1 H NMR and IR spectra?
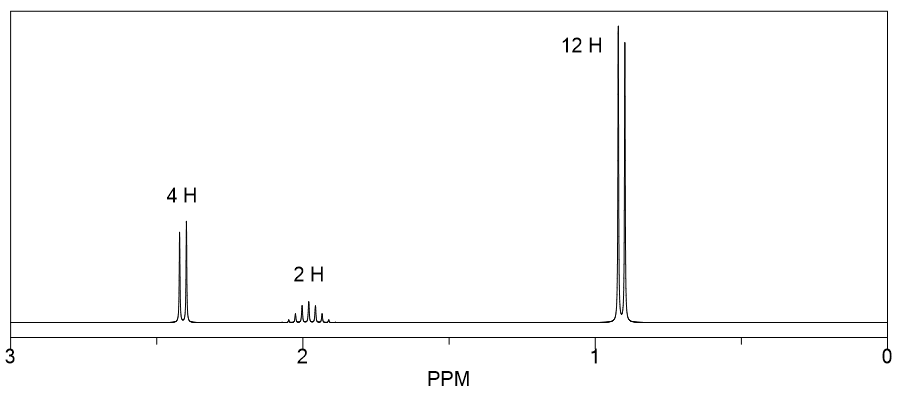
The IR spectrum of compound A with the molecular formula C 3 H 6 O is shown below.
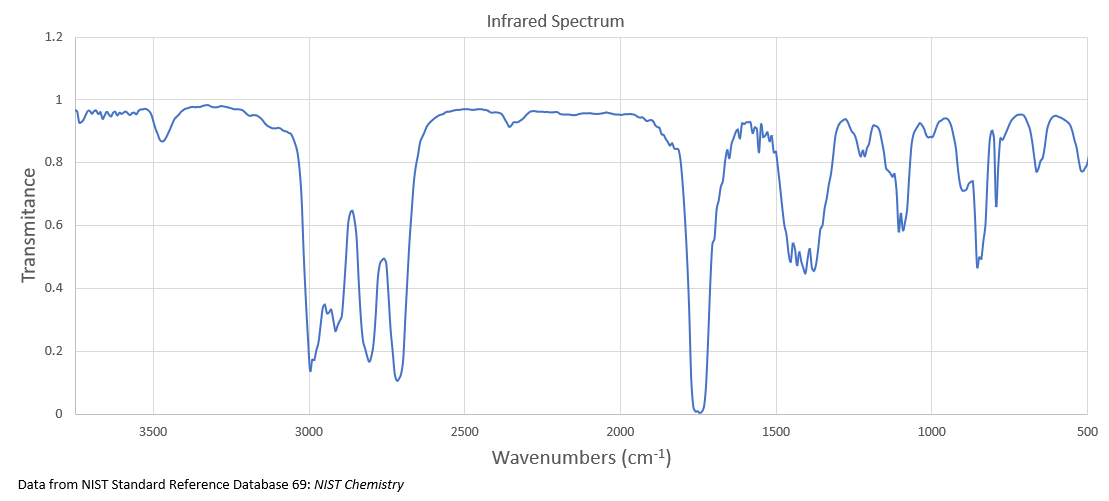
It undergoes reduction to produce compound B whose 1 H NMR spectrum is displayed below.
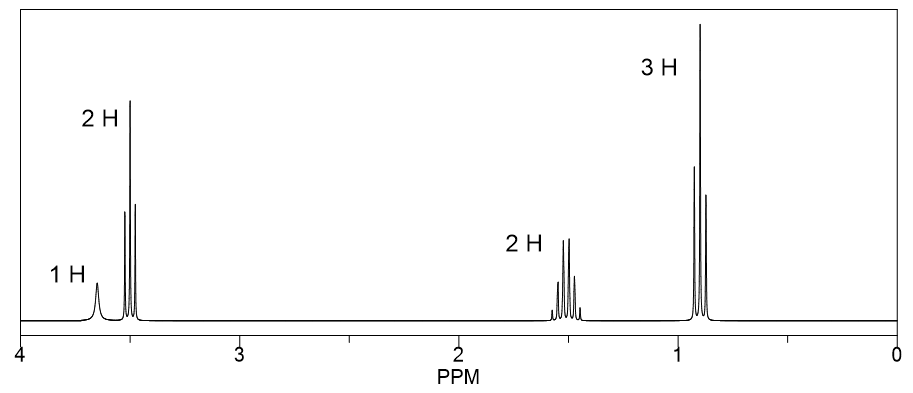
Determine the structure of the compounds.
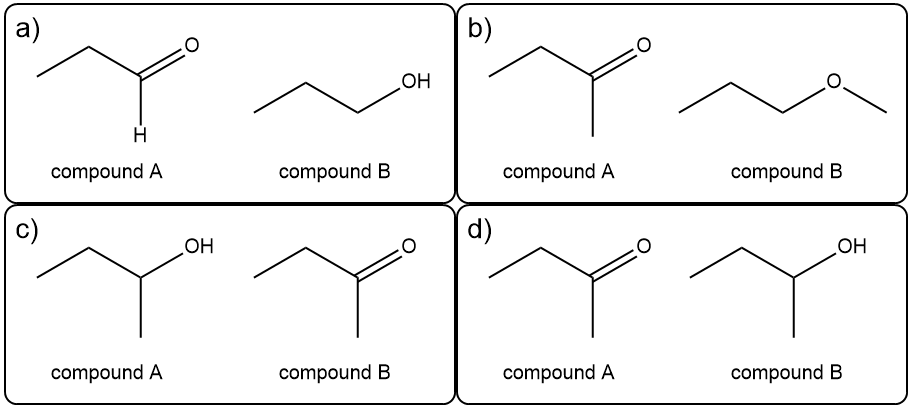
Draw the most probable NMR spectra for the compound below:
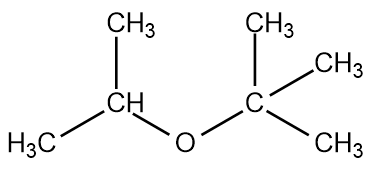
Draw the possible NMR spectra for the compound below:

Using the proton NMR spectra specify the characteristic difference in peaks between the pair of compounds in each of the following.
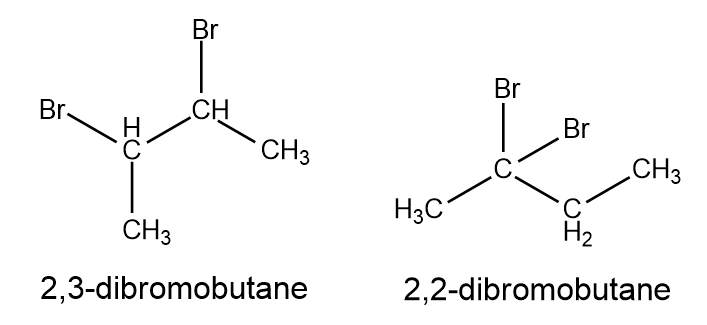
Predict the possible NMR spectra for the molecule below.
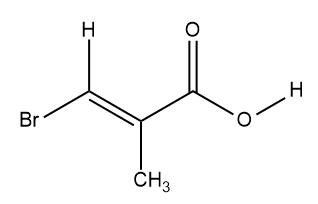
Below are characteristic proton NMR signals for each of the four structures. Each has the molecular formula C 6 H 12 O 2 . Match the correct proton NMR signals with their corresponding structure.

(I) 3H triplet at 1.1 ppm and 2H quartet at 2.3 ppm
(II) 6H doublet at 0.9 ppm and 3H singlet at 3.7 ppm
(III) 6H doublet at 0.9 ppm and 1H broad singlet at 11.0 ppm
(IV) 3H singlet at 2.3 ppm and 1H broad singlet at 3.6 ppm
Propose a structure of the compound with molecular formula C 5 H 12 O that gives the following NMR spectrum. Assign the molecule's protons, giving rise to labeled peaks in the spectrum.
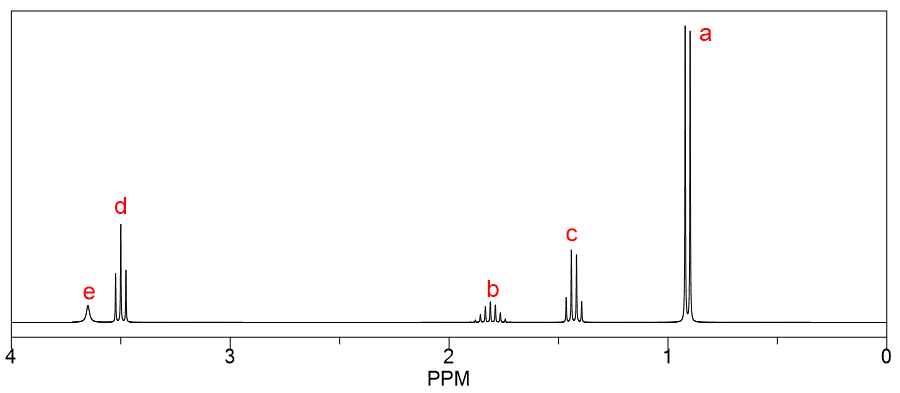
One Strong and another medium IR absorption at 1650 cm −1 and 1700 cm −1 were observed for an unknown compound with molecular formula C 3 H 3 BrO. The proton NMR spectrum of the compound shows two doublets at 6.7 ppm and 7.3 ppm, respectively, with J = 14 Hz and one singlet at 9.7 ppm. Predict the structure consistent with the above information.
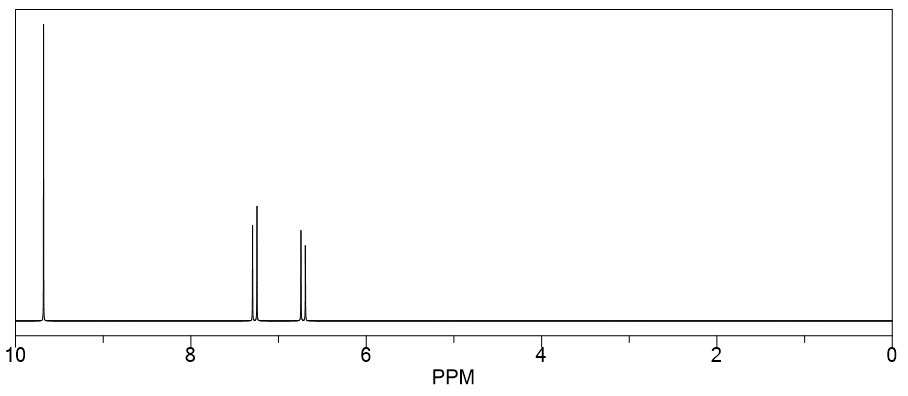
Determine the structure for the following NMR spectrum and molecular formula. Also, state the type of protons that give the peaks at 2.5 ppm and 2.7 ppm.
Molecular Formula: C 6 H 10 O 3
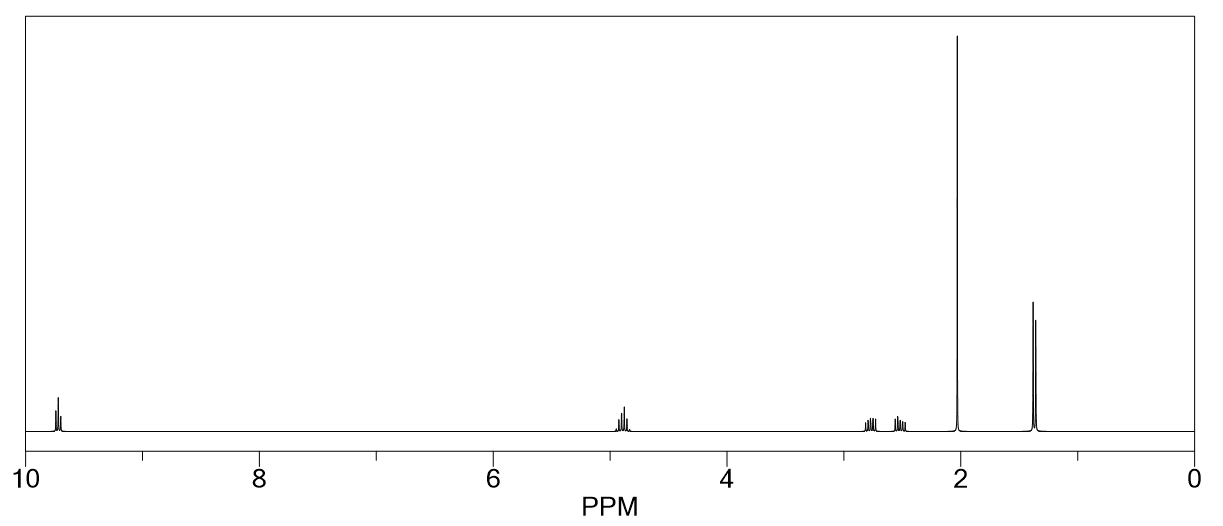
Predict the spectral assignments for the protons in 3-methylbutan-2-ol along with the multiplicity and integration. Note: Integration refers to area or number of protons corresponding to a particular peak.
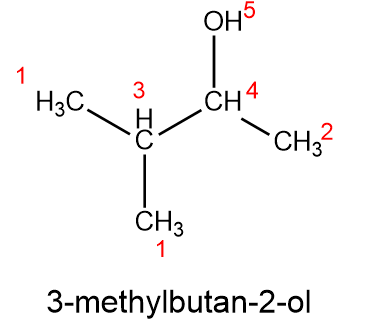
A proton NMR spectrum along with the molecular formula is given below. Identify the structure that is consistent with the given NMR spectrum and molecular formula.
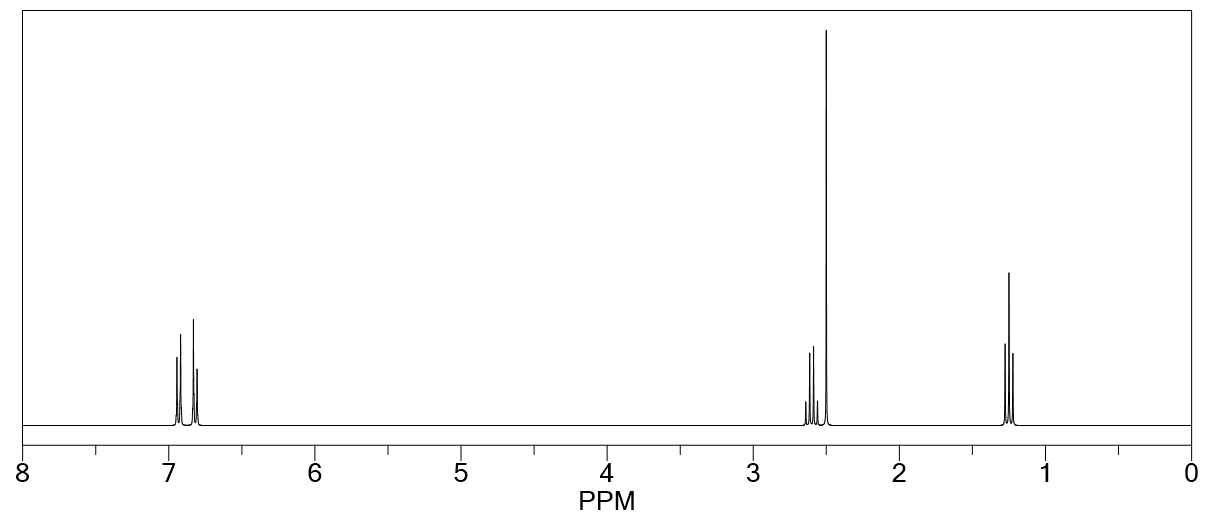
Proton NMR spectrum of an unknown compound with molecular formula C 13 H 13 BrO 6 has three singlets:
At 3.9 ppm for 9H
At 4.6 ppm for 2H
At 8.1 ppm for 2H
Identify the most probable structure for this compound.
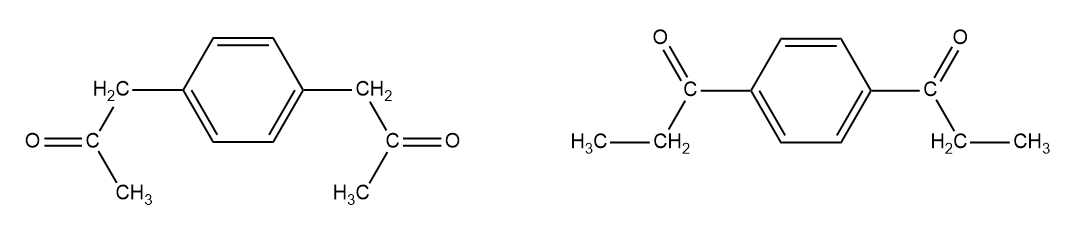
Identify the NMR spectrum for ethyl isobutyrate from the options below and identify the two main peaks that make it different from the spectrum of isopropyl propionate.
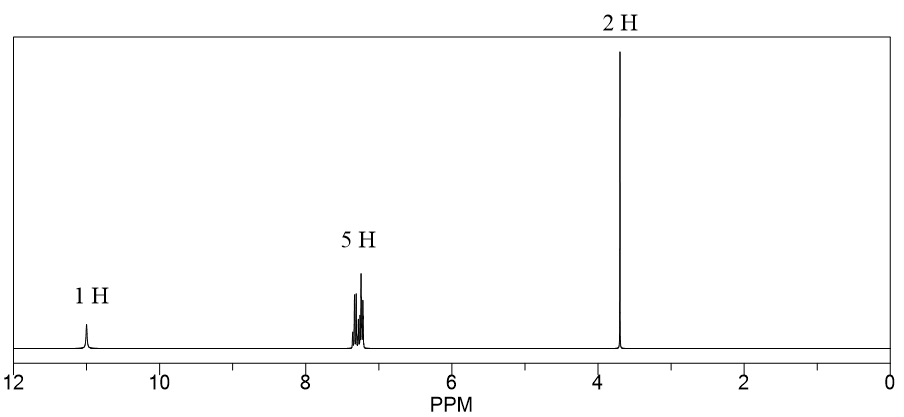
The OChem Whisperer
Guide to Solving NMR Questions
How to solve any nmr question.
Solving NMR questions is easier than you think. All you need is a step-by-step process to help guide you through each question. And here it is…
Most NMR questions on an exam involve determining a specific structure rather than memorizing and repeating various NMR values. Typically, you will be given an NMR spectra and a molecular formula (sometimes an IR spectra will be provided). I have put together a few ideas that might make this process a bit easier. I am in the process of putting together a more concise document than this as a study aid. This post is meant to walk you through the thought process of how to tackle this type of problem. The description is a bit long (….so hold on!), but once you get it, you can just use the algorithm to solve your NMR problems. Here are some reference values and a couple of proton NMR spectra:
Proton NMR Reference Values

(cem.msu.edu)

(process-nmr.com)

(Our example 1H NMR spectra for this post; unknown source)
Start with an algorithm to get you on track
When staring an NMR question, you can use the following algorithm to help guide you through the thought process:

(above should say C2H5Cl = C2H6)
Calculate Degrees of Freedom
We notice the first thing says calculate degrees of unsaturation… what is that ? This allows us to determine if there are any double bonds or rings (cyclic structures) in the compound. Let’s look at an example; the formula is C 4 H 8 O 2 . Now, we need to compare this formula with the formula of a completely saturated hydrocarbon (all single bonds…no double bonds):
This formula tells us how many hydrogens we need to have a carbon compound with NO double bonds or rings. Let’s look at our example…
If I have a compound with 4 carbons, this 4 refers to n. Now, if we plug this in the formula, we get
C 4 H 2(4)+2 = C 4 H 10
This says that if we have a compound with only 4 carbons, we need 10 hydrogens to have a compound with no double bonds or rings. If we look at our example, we have C 4 H 8 O 2 . For now, all we need to look at is the C 4 H 8 when dealing with degrees of unsaturation (we will discuss what to do with heteroatoms a bit later). What we now want to do is subtract the hydrogens in our example ( C 4 H 8 ) from the saturated formula ( C 4 H 10 ):
C 4 H 10 – C 4 H 8 = 2 hydrogens
This leaves us with a value of 2. Now, the last things we do to get our degrees of unsaturation is divide this number by 2:
How to use the degrees of unsaturation to get the answer
This leaves us with 1; therefore, we have 1° of unsaturation. So, what does this mean? Each degree of unsaturation equates to a double bond or ring. Here are a few examples to further clarify:
1° of unsaturation = 1 double bond or 1 cyclic structure
2° of unsaturation = 2 double bonds; 1 alkyne; 1 double bond and 1 cyclic structure; 2 cyclic structures
3° of unsaturation = 3 double bonds; 2 double bonds and 1 cyclic structure; etc…
When you see 4° of unsaturation, think benzene; 3° of unsaturation for the 3 double bonds and 1° of unsaturation for the ring.
In our example, it means we have one double bond or one cyclic structure in our compound. Let’s look at few ring systems:

The larger the ring, the more stable the ring (with this series). Three and four-membered rings are rare. Usually, you will hear more about 5- and 6-membered rings. Since this is the case, we more than likely have a double bond (but never rule out a ring until you have looked at the NMR spectra).
Next, if we look at the algorithm, we need to consider the other atoms (other than carbon and hydrogen) in our formula…oxygen. Since we have degrees of freedom…think carbonyl. That is all we can do for now with our algorithm. Let’s now look at our spectra and see if we can start to determine the structure from the peaks:
Interpreting the spectra – splitting patterns

We have three peaks: a quartet around 4 ppm; a singlet around 2 ppm; and a triplet around 1 ppm. At this point, let’s review singlet, doublet, triplet, quartet, and multiplet:

What do these peaks refer to and how to we get the specific peak pattern? Well, we use the n+1 rule to figure out the pattern:

Putting the fragments together
Once you have your fragments, it is a matter of figuring out how to put them together. By looking at the spectra and where the peaks show up (ppm), you can figure out how the fragments go together.

Click NMR pictures to see the images as a PDF.
Don’t forget IR Spectroscopy!
Here is a simple guide showing you the most common IR values
Share this:
Leave a reply cancel reply.
Your email address will not be published. Required fields are marked *
Notify me of follow-up comments by email.
Notify me of new posts by email.

Chemistry Steps

Organic Chemistry
Nuclear magnetic resonance (nmr) spectroscopy.
At times solving an NMR problem leads to two or more plausible structures satisfying the given data. We have seen that 13 C NMR is usually decoupled and therefore there is no splitting of signals which limits the information we can get as to how many hydrogens are connected to a carbon atom.
And even combining 1 H and 13 C NMR spectra may not give definite evidence for choosing only one structure.
This is where a technique called DPET ( distortionless enhancement by polarization transfer ) becomes very useful. All it does (and that’s a lot and very handy) is it differentiates the carbons based on the number of hydrogens it is bonded to.
So, instead of simply saying hey this is a carbon, and this is another one, it tells us if it is a C, CH, CH 2 , or a CH 3 . Isn’t that nice?
Let’s see how we get this information in DEPT.
Depending on the carbon type, the signal in DEPT can be pointing up or down while being at the same ppm values as in the regular 13 C NMR. Another possibility in DEPT is the lack of a given signal . Here is the summary of DEPT signals :

DEPT-90 and DEPT-135 are different types of DEPT experiments and we won’t go over the mechanisms here but rather use this data as it is. The aim of this article is to explain the application of DEPT in solving NMR spectra .
As an example , let’s see this (stimulated) 13 C NMR combined with the DEPT experiments:

Notice how the ppm values are retained but depending on the signals in DEPT we can tell if the carbon is a C, CH, CH 2 or a CH 3 group .
In case you needed, here are the chemical shift values for 13 C NMR:

Show Me a Good Example of DEPT NMR Problem
Let’s discuss a specific NMR problem where the final structure is only determined using the DEPT data.
The proton and carbon NMR spectra of a compound with the formula C 5 H 9 Br are shown below. The DEPT experimental results are also provided in the table.

Purpose a plausible structure based on the data provided.
I went over the steps for solving NMR problems with lots of examples which you can find here but for now let’s quickly apply those and see what we get.
First, determine the hydrogen deficiency index . Replacing the Br with an H we get C 4 H 10 which corresponds to one degree of unsaturation . Therefore, the compound has a double bond or a ring .
Now, looking at signal at about 4.7 ppm in the proton, and the ones above 100 on the carbon, we know that it must be a double bond rather than a ring.
Next, look at the signal splitting in 1 H NMR; two triplets indicate a -CH 2 -CH 2 – fragment which is connected to Br on one end since it is downfield (3.3 ppm) . And the other CH 2 must be connected to the double bond since the signal is still more downfield than if it was a regular alkyl group.
I’ll put this table for 1 H NMR shifts for a reference:

So, let’s put down the groups we have so far:

Two of these X groups must be hydrogens because of the integration of the signal at ~4.7 ppm.
The other X group is a methyl group which we can deduce from the integration.
And now the i nteresting part realted to DEPT . There are three combinations of putting two hydrogens and a methyl group on the double bond:

All of these would be good candidates based on the data from the proton and carbon NMR. But only the last structure matches the data from the DEPT experiments which indicate the presence of three CH 2 groups (three negative signals in DEPT-135):

I do want to mention that the structure of a double bond can be analyzed using the J coupling values and a powerful NMR spectrometer will give a resolution good enough to exclude the other candidates based on the coupling. However, DEPT makes things easier without the need for a lot of complicated analysis.
- NMR spectroscopy – An Easy Introduction
- NMR Chemical Shift
- NMR Chemical Shift Range and Value Table
- NMR Number of Signals and Equivalent Protons
- Homotopic Enantiotopic Diastereotopic and Heterotopic
- Homotopic Enantiotopic Diastereotopic Practice Problems
- Integration in NMR Spectroscopy
- Splitting and Multiplicity (N+1 rule) in NMR Spectroscopy
- NMR Signal Splitting N+1 Rule Multiplicity Practice Problems
- 13 C NMR NMR
- DEPT NMR: Signals and Problem Solving
- NMR Spectroscopy-Carbon-Dept-IR Practice Problems
1 thought on “DEPT NMR: Signals and Problem Solving”
Leave a comment cancel reply.
Notify me of followup comments via e-mail. You can also subscribe without commenting.
Have we been helpful? Please let us know in the Reviews section here.
WassUp 1.9.4.5 timestamp: 2024-05-29 08:53:32PM UTC (03:53PM) If above timestamp is not current time, this page is cached.
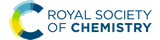
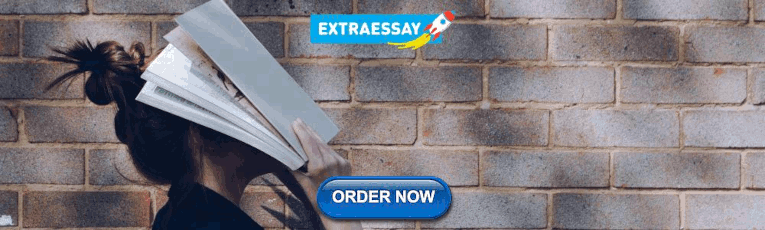
Support for instructional scaffolding with 1 H NMR spectral features in organic chemistry textbook problems

First published on 24th March 2020
Nuclear magnetic resonance (NMR) spectroscopy is vital to synthesis and provides rich problem-solving opportunities to organic chemistry students. Using the theories of scaffolding, interleaving, and blocking, our research systematically explores how textbooks introduce and reinforce spectral features when teaching students to solve 1 H NMR spectroscopy problems. Specifically, we investigated the 1 H NMR spectral features presented in worked examples and practice problems across four undergraduate organic chemistry textbooks. We examined the frequency and ordering of spectral features to explore how the textbooks could support scaffolded instruction. Spectral features like the number of signals and chemical shift were covered by problems more frequently, while integration was covered least. Our findings suggest that textbooks do not provide sufficient practice with all 1 H NMR spectral features. We observed no discernible pattern in how textbooks ordered spectral features of 1 H NMR in problems, indicating that there is little systematic method to the design of textbook chapters. Implications for textbook authors and editors, instruction, and research are discussed.
Introduction
As a result of NMR spectroscopy's importance to chemists and its vital role in synthesis, interpreting these spectra and identifying unknown compounds with spectra serve as a component of organic chemistry instruction. In undergraduate organic chemistry, students learn about both 1 H and 13 C NMR spectroscopy, along with other spectroscopic and spectrometric methods. While all methods can be used together in the characterization of organic compounds, 1 H NMR spectroscopy provides enough information by itself to deduce the whole structure of an unknown organic compound. Instructors often note—anecdotally—that students struggle with 1 H NMR spectroscopy, but systematic research exploring these concerns is lacking. Research on student understanding of NMR spectroscopy has focused on the characteristics of graduate-level solvers ( Bodner and Domin, 2000 ; Cartrette and Bodner, 2010 ; Domin and Bodner, 2012 ) and on how undergraduate students approach such problems ( Topczewski et al. , 2017 ; Connor et al. , 2019 ; Stowe and Cooper, 2019 ).
While solving 1 H NMR spectroscopy problems, undergraduate students have been shown to make incorrect assumptions about spectral features and resort to heuristics to make their interpretations ( Connor et al. , 2019 ). While students have been shown to possess adequate procedural knowledge on approaching 1 H NMR spectroscopy problems, they have been found to lack reasoning to support their answers ( Stowe and Cooper, 2019 ). Likewise, it has been shown that undergraduate students do not spend their time solving by connecting spectral data with their answers; students lack the checking procedures that experts utilize ( Topczewski et al. , 2017 ). Connor and Shultz (2018) examined the pedagogical content knowledge (PCK) in 1 H NMR spectroscopy with teaching assistants and found that PCK increased with experience in teaching the subject of NMR. Additionally, they found that TAs struggled to identify what would make similar problems difficult and to provide teaching strategies for those problems, indicating that PCK with NMR may be specific to certain problems and topics. From these studies, we see that undergraduate students need further instruction and opportunities on checking and supporting their answers when solving 1 H NMR spectroscopy problems, and extended coverage on each of the spectral features to improve their interpretations of spectral data and prevent heuristic use. These studies have examined students solving spectroscopy problems and provide insight in the area of teaching NMR, but to our knowledge no studies have analyzed how the content of 1 H NMR spectroscopy is presented to students.
Textbooks are a reliable resource for learners, created by experts in the field, assigned by professors, and made available to all students. A number of studies have examined undergraduate chemistry textbooks, and have shown that textbooks are important to teaching and learning chemistry ( Gkitzia et al. , 2011 ), often guiding the organization of a course's curriculum ( Koppal and Caldwell, 2004 ). Just as different general chemistry textbooks have been shown to present the same concepts differently in the narrative ( Pyburn and Pazicni, 2014 ), in practice problems ( Dávila and Talanquer, 2010 ), and with representations ( Nyachwaya and Gillaspie, 2016 ), we can likewise discern and establish the different ways students are being presented with content on 1 H NMR spectroscopy. We therefore focused our research on the potential of textbooks to support instruction on 1 H NMR spectroscopy.
Instructional scaffolding can support the learning of spectroscopy
While scaffolding holds promise in enabling students to master activities such as problem solving with spectroscopy, the term scaffolding itself has been interpreted and used in different ways. Research has depicted scaffolding as involving an instructor providing structures to support a student's performance ( Wood et al. , 1976 ; Rosenshine and Meister, 1992 ), becoming a participant in a student's learning process ( Bruner, 1983 ; Mercer and Littleton, 2007 ), helping a learner accomplish a task that they would not have been able to accomplish on their own ( Bruner, 1974 ; Maybin et al. , 1992 ), and systematically devising the sequencing of prompted content, tasks, and support to optimize student learning ( Dickson et al. , 1993 ). Most simply stated, scaffolding is a support for learning (as inferred from its metaphor to construction). However, descriptions of scaffolding in practice—and what it looks like in the classroom—are limited. Synthesizing research on scaffolding, van de Pol et al. (2010) proposed a framework to characterize instructional scaffolding. Drawing from research by Tharp and Gallimore (1988) and Wood et al. (1976) , van de Pol et al. (2010) describes a scaffolding strategy as involving two dimensions, means and intentions ( Table 1 ). The means of a scaffolding strategy include clear pedagogical moves like providing feedback or demonstrating a particular skill. The second dimension of a scaffolding strategy is the intention, that is the purpose of the scaffolding strategy. The intention can include actions that help the student stay on task or organize their thinking. van de Pol et al. (2010) proposed that scaffolding is comprised of both an intention and means, where the means of scaffolding act to implement the intentions of scaffolding. For example, an instructor may want to aid a student in staying on task toward the current goal (direct maintenance) by the act of asking the student a question (questioning).
Although the broad use of the term scaffolding has been criticized for being vague and meaning nothing more than support ( Pea, 2004 ; Puntambekar and Hubscher, 2005 ), the framework of scaffolding as proposed by van de Pol et al. (2010) addressed these criticisms by clearly defining the intentions and means of scaffolding strategies in instruction. We propose that scaffolding is essential to learning 1 H NMR spectroscopy because the task is complex, with multiple steps and spectral features dependent on the diverse characteristics of a compound. As shown by van de Pol et al. , scaffolding strategies can be infused at different curricular levels, such as the course, unit of instruction, or even the individual item. In fact, Stowe and Cooper (2019) investigated scaffolding at the item level by guiding students’ argumentation to different extents through item prompts. They found that scaffolding at the level of the prompt showed no impact on students’ abilities to construct evidence-based arguments behind their 1 H NMR spectroscopy problem solving. We hypothesize that learning 1 H NMR spectroscopy could benefit from scaffolding on the level of the unit as a whole, where intentions like reducing the degrees of freedom would provide students with problems requiring the use of the chemical shift, before encountering problems requiring the use of the chemical shift and the splitting.
Undergraduate organic chemistry students learn about several spectral features (in particular, the number of signals, chemical shift, integration, and splitting), are expected to correctly interpret those features and then use those spectral features together to determine the structure of the compound represented. With intentions (or goals) like direct maintenance, focused cognitive structuring, and reducing the degrees of freedom, an instructor can directly reduce the cognitive demand on a student while interpreting 1 H NMR spectra. Furthermore, through means such as instructing, explaining, and modeling, an instructor can show a student how to approach their interpreting of 1 H NMR spectra.
Textbooks are reliable instructional tools that can support scaffolded instruction
Although textbooks are resources unto themselves, scaffolding is a process between the instructor and student. Therefore, textbooks have the potential to support instructional scaffolding, but how textbooks function as part of the scaffolding process is highly instructor-dependent. For example, an instructor may use a textbook to introduce spectroscopy before going over the information in class. An instructor may also have students refer to the textbook upon introducing the subject in class, where the textbook provides students with clarification and practice on the task. An instructor may also use the textbook problems, both within the chapter and at the end of the chapter, as formative or even summative assessment. Regardless of what an instructor chooses to do, textbooks have the potential to explicitly support scaffolded instruction in the areas of cognitive structuring and reducing the degrees of freedom through content that explains and models , and can fade that support through the order and structure of problems ( van de Pol et al. , 2010 ).
Learning to interpret 1 H NMR spectra is a complex task, one that benefits from instructional scaffolds. Students must be able to comprehend and interpret each spectral feature, use all the spectral features together, and be able to move forward or backward in that interpretation process (either by predicting what a spectrum should look like based on the compound or by inferring what is represented by a given spectrum). Ideally, textbooks should provide material sufficient for mastering all areas of interpretation. Specifically, textbooks should provide support for cognitive structuring with features to instruct and explain each spectral feature of 1 H NMR spectroscopy and then reduce the degrees of freedom by modeling areas of interpretation. Building student understanding of these spectral features one at a time, then moving to two or three spectral features together, before moving to all four spectral features together slowly introduces more degrees of freedom into the problem-solving process. Once all spectral features have been practiced alone and together, practice problems can fade the support further when the arrangement of problems does not foreshadow the approach to take. For example, an organic chemistry textbook could provide examples and questions for the number of signals, chemical shift, integration, and splitting individually and then together, modeling how to use all the spectral features alone and in concert to interpret a full spectrum. Worked examples and practice problems allow textbooks to accomplish this support to instructional scaffolding.
Worked examples and practice problems demonstrate potential support for instructional scaffolding
Reducing cognitive load, worked examples can help students in identifying how and why steps are taken in a path to solve a problem by allowing them to focus exclusively on the characteristics of that path, rather than both creating a solution path de novo and then evaluating the accuracy or correctness of that path. For the novice solver, evaluating the accuracy or correctness of a path may be cognitively taxing. Evidence from mathematics and the learning sciences demonstrates that worked examples can reduce cognitive load ( Cooper and Sweller, 1987 ; Paas, 1992 ; Paas and van Merriënboer, 1994 ; Paas et al. , 2016 ; van Gerven et al. , 2002 ). Moreover, reducing cognitive load functions analogously to the reduction of the degrees of freedom in scaffolding. In 1 H NMR spectroscopy, worked examples can reduce cognitive load by allowing the learner to focus on the interpretations that can be made with each individual spectral feature and then use all the spectral features together to come to a solution.
In contrast, practice problems ( Fig. 1B ) provide a problem statement, but do not provide the answer or goal state. Left as an unknown, students must have a developed problem-solving approach to find the goal state. Thus, practice problems have a very different learning goal, namely to provide students with opportunities to practice, refine, and expand problem-solving approaches. Practice problems serve as an implicit part of the scaffolding process because they do not explicitly indicate which spectral features to use when solving a problem. Practice problems support scaffolded instruction as a diagnostic that addresses areas in need of continued cognitive structuring, and serve as a form of faded support that increases the degrees of freedom. Moreover, the systematic order and combination of problems can implicitly function as a means of scaffolded instruction.
The sequencing of practice problems through interleaving and blocking can impact scaffolding
The interpretation of spectral features to solve 1 H NMR spectroscopy problems may not be directly comparable to the problem-solving approaches in the literature on interleaving and blocking, but the material could benefit from interleaving. In 1 H NMR spectroscopy, the strategy or approach does not change between problems: as students interpret spectra, they should attend to all four spectral features each time. However, in each problem, a specific spectral feature or a few spectral features may lead to the structure solution; those spectral features vary with each problem. Additionally, the order in which a solver attends to each spectral feature may differ with the problem and/or the person solving. No matter the order used in solving the problem, the student should still use and interpret all four spectral features. Finally, while there is no evidence that students confuse one spectral feature of 1 H NMR spectroscopy with another, interleaving could still be a useful tactic to help students discern how and when to use the spectral features and make the practice problems more diagnostic in nature, so a student knows which spectral feature(s) they do not understand.
Outside the context of categorization and identification tasks, we hypothesize that blocking and interleaving could work together to promote mastery in problem solving. Blocking supports the mastery of a single spectral feature, while interleaving supports mastery of the overall problem-solving process. Blocking can function to aid the student in mastering the interpretations and uses of specific spectral features, and with support from the text, blocking can reduce the degrees of freedom regarding the determination of which spectral features to pay attention to. Students can, therefore, focus on mastering interpretation with one spectral feature at a time. Moreover, interleaving allows for further mastery of problem solving by fading that support, letting students develop and evaluate the solution pathway. While research on interleaving has purported the use of interleaving over blocking, according to van de Pol's framework, the intentions of scaffolding apply to both blocking and interleaving. Thought should go into using both blocking and interleaving to build the problem-solving pathway.
Through the ordering of spectral features within worked examples and practice problems found in organic chemistry textbooks, students are provided with multi-faceted opportunities to develop their knowledge of 1 H NMR spectroscopy and problem solving. Systematically examining the worked examples and practice problems for the presence of these spectral features of 1 H NMR spectroscopy provides evidence of how textbooks can support the scaffolded instruction of 1 H NMR spectroscopy. In this study, we characterized the potential for supporting scaffolded instruction in worked examples and practice problems found in organic chemistry textbooks commonly used in undergraduate instruction. Specifically, we asked:
1. What 1 H NMR spectral features do textbook worked examples and practice problems focus on?
2. How do textbook worked examples and practice problems show evidence of interleaving and blocking with 1 H NMR spectral features?
Textbook selection and sampling
Coding and analysis.
To determine which 1 H NMR spectral features were the focus of worked examples and practice problems, each problem and worked example was coded for the specific spectral feature(s) indicated by the problem. Those spectral features were the number of signals/proton equivalency (#S), chemical shift (CS), integration (INT), and splitting (SPL). The number of signals in the spectrum indicates the number of equivalent proton groups the compound has and is equal to the number of different types of protons. The chemical shift , or the position of a signal, is determined by shielding and deshielding effects, where shielding shifts an absorption upfield and deshielding shifts an absorption downfield. Integration is the area under a signal and is proportional to the number of absorbing protons. Splitting , the pattern of absorption peaks resulting from spin–spin splitting of nuclei, can be used to determine how many protons reside on the carbon atoms near the absorbing proton. The number and nature of adjacent protons determines the observed splitting pattern. These four spectral features were examined because they encompass the information on 1 H NMR spectroscopy most readily and universally available in undergraduate organic chemistry instruction. While some undergraduate courses may cover spectral features like spin–spin coupling and diastereotopic protons, the four spectral features we examined are because they pertain to a baseline of features covered in undergraduate instruction. An a priori coding scheme of the spectral features was established by two researchers, and one researcher independently coded all worked examples and practice problems. The second researcher took a random sample of worked examples and practice problems, comprising 25% of the total sample, and coded them to establish reliability (Cohen's kappa: 0.945).
Once the practice problems and worked examples were coded for the four outlined 1 H NMR spectral features (see example in Fig. 2 below), the scaffolding of problems was examined in terms of the ordering and combinations of those spectral features. The ordering of the spectral features for the in-text practice problems and worked examples was compared to the ordering of the spectral features in the narrative. To support our goal of identifying evidence of blocking and interleaving, we used sunburst diagrams to visualize the spatial distributions of spectral features in worked examples and practice problems. We modified a typical sunburst diagram to show the progression of spectral features used in worked examples and practice problems.
Coverage of spectral features
All four examined spectral features were featured in the practice problems in all textbooks, both in the in-text and end-of-chapter practice problems ( Table 6 ). The number of signals and chemical shift were the most frequent spectral features among the textbook problems. Among the practice problems, integration was featured the least, comprising of 55% of the problems on average across the textbooks.
We also explored the number of problems targeting single or multiple spectral features ( Table 7 ). We found that, in general, worked examples were more likely to focus on a single spectral feature at a time, while practice problems focused on all four spectral features together.
Ordering of spectral features
A sunburst diagram is read in a clockwise manner, starting at the topmost notch in the circle. The gray ring indicates the page on which a particular problem was found. Each colored ring conveys the chronology of a different spectral feature within the textbook. For example, pink denotes number of signals, and the light pink signifies the spectral feature was observed in a worked example and dark pink in a practice problem. This convention is used for each spectral feature: blue denotes chemical shift, green denotes integration, and purple denotes splitting. If no color is present in a portion of the ring, the spectral feature for that respective ring was not exercised by the problem in question. Pages without any colored rings indicate a page with no problems.
Sunburst diagrams support our exploration of blocking and interleaving of spectral features within worked examples and practice problems in both within-chapter and end-of-chapter problems. Given our definition of interleaving as the deliberate intermixing of spectral features, we would not expect to see solid rings of color in our sunburst diagrams, but we would see different colors mixed across the problems. With interleaving, we would not see multiple problems of a spectral feature in a row, as that would signal to a student that that is the feature to use. In contrast, if blocking provides a set of problems grouped together that exercise the same spectral feature(s), we would expect to see solid rings of color as evidence of blocking of spectral features.
We see little evidence of blocking or interleaving in the Brown et al. within-chapter problems. Rather, we see some evidence of an additive approach, where the problems introduce one spectral feature and add subsequent spectral features in turn.
The end-of-chapter problems in Brown et al. almost exclusively focus on the use of all four spectral features. There is no evidence of interleaving, and the use of all four spectral features in the bulk of problems shows evidence of poor scaffolding.
We see evidence of blocking in the within-chapter problems of Carey and Giuliano, with sets of problems devoted to chemical shift, then the number of signals, and later with the number of signals and splitting together. Again, the focus is primarily on one spectral feature at a time and no worked examples or practice problems target all four spectral features together.
The end-of-chapter problems begin with two spectral features, the chemical shift and number of signals, but expand to focus on all four spectral features at a time. Several problems then focus on chemical shift and integration, followed by a single problem on only splitting, and then a set of problems on all four spectral features again. At the end of the chapter narrative, the problems focus on random spectral features and combinations of spectral features before going back to all four spectral features together. We see little evidence of interleaving in the end-of-chapter problems, but there is evidence of blocking, followed by a set of problems with all four spectral features. While the ordering of the spectral features becomes more random toward the end of the problem set, most problems are focused on using three or four spectral features simultaneously.
With the few within-chapter problems it is difficult to make claims about blocking or interleaving. There is some blocking toward the end of the section. One practice problem in the middle of the chapter targets all four spectral features, while no worked examples target all four spectral features together.
We see evidence of an additive approach to the spectral features in the end-of-chapter practice problems for Jones and Fleming, starting with the number of signals, then moving to chemical shift, and then chemical shift and integration before focusing on all four spectral features together. These problems show evidence of blocking. The ordering of the spectral features gets more unpredictable in the last two-thirds of the problems, focusing several problems on chemical shift then all spectral features together except the number of signals. More random combinations of spectral features are targeted before focusing primarily on all four spectral features together at the end. These random combinations could be possible evidence of interleaving, but with blocking throughout the problem set, the evidence for interleaving is not strong.
We see evidence of blocking with Smith's within-chapter problems. Overall, the within-chapter problems focus on a single spectral feature at a time throughout much of the chapter; some of the later problems incorporate three or four spectral features, but the spectral features are built up. This additive approach to the spectral features is shown as Smith focuses on the first two spectral features independently and then together. This building is repeated for a fourth spectral feature with a set of problems using only splitting, then using splitting with the three other spectral features. There is no evidence of interleaving.
We see evidence of blocking in the end-of-chapter problems, where a series of problems on the number of signals are given, followed by the number of signals with integration. Chemical shift problems are then provided, followed by problems with the number of signals and splitting. A series of problems on splitting are provided before problems are given on all four spectral features together. Each spectral feature is handled independently before all four spectral features are used together. There is no evidence of interleaving with the Smith end-of-chapter problems.
Across all four sampled textbooks, the ordering and combinations of within-chapter problems varied. Where Brown et al. (2008) utilized an additive approach, beginning with one spectral feature then adding another spectral feature to that previous spectral feature, Carey and Giuliano (2011) focused on each spectral feature independently. Jones and Fleming (2014) moved back and forth from combinations of spectral features, and Smith (2011) was additive with spectral features, but also provided multiple practice problems and worked examples with all four spectral features together. In the end-of-chapter problems it is evident that all four spectral features together are utilized in all the textbooks, but the textbooks’ approaches still differ. Brown et al. (2008) almost exclusively focuses on all four spectral features together, Carey and Giuliano (2011) offer a few problems with all four spectral features in the middle of the problem set and then follows with problems with fewer spectral features and repeating this process. Jones and Fleming (2014) starts out with an additive approach and then gets more random in the combinations of spectral features, while Smith (2011) covers each spectral feature alone before combining multiple features and ending with all four spectral features together. In a full comparison, all four textbooks continue to vary in their approaches. Between all the worked examples and practice problems (within-chapter and end-of-chapter), three of the textbooks showed evidence of blocking (Carey and Giuliano, Jones and Fleming, and Smith). Three textbooks showed some additive approaches with the spectral features (Brown et al. , Jones and Fleming, and Smith). Only one textbook showed potential for interleaving (Jones and Fleming).
Individual spectral features
Without adequate practice with each individual spectral feature, textbooks could be shortchanging the learning process for students. As in many of the textbooks we chose not to include in this study, when there are few worked examples or practice problems on specific spectral features, it can impact the development of fluency in problem solving. Students working with a limited number of problems for a particular spectral feature will not see the diversity in problems and gain the fluency and flexibility necessary in using the spectral features together. As Stowe and Cooper (2019) observed, students do not build evidence-based arguments to support the answers they arrived at. We would argue that textbook coverage of spectral features could build problem solving fluency and by extension, improve students’ argumentation. Furthermore, a lack of worked examples and practice problems could lead students to adhere to heuristics like generalization and rigidity, and students could make assumptions that spectral data should be absolute ( Connor et al. , 2019 ). The more problems students have access to, the more ways they can observe spectral features being used, which could help them build fluency in using those spectral features.
In our analyses, we found that integration comprised 55% of the practice problems on average across the textbooks, the least of all the spectral features. Students may implicitly rely on those spectral features that are overrepresented, mistakenly believing those are key spectral features for solving problems. By extension, students do not develop a robust problem-solving approach. Without adequate practice on integration, a student could be uncertain in interpreting the number of hydrogens represented by individual signals sets and could therefore fail to see how to connect the structural pieces together. Each 1 H NMR spectral feature is important to the solution pathway. With some spectral features overrepresented in worked examples and practice problems, a student may develop an understanding that those lesser assessed spectral features are not as important to the problem-solving process. While it could be argued that some spectral features may be more foundational than others and therefore deem more practice, it is not necessarily the role of a textbook to determine what is needed most by students and a textbook should not signal what could be arguably more foundational—this is a role of the instructor. In their use of textbooks, instructors make decisions on what to use, supplement, and alter from a textbook ( Mesa and Griffiths, 2012 ). Likewise, students search out examples in their use of textbooks ( Weinberg et al. , 2012 ; Lee et al. , 2013 ). As sufficient support for scaffolded instruction, textbooks could instead include bountiful examples and problems of all types that an instructor can refer their students to.
Support for instructional scaffolding by combining spectral features
Worked examples and practice problems support instructional scaffolding by giving learners many problems and examples involving each individual spectral feature prior to encountering a problem involving all the spectral features. If textbooks are to support instructional scaffolding in the learning process, we would expect to find worked examples and practice problems that examine each spectral feature in isolation as well as in combination to assess students’ ability to interpret and use multiple spectral features. Students could benefit from seeing additional worked examples that combine spectral features to better learn how the four spectral features work in concert to solve a 1 H NMR spectroscopy problem. The novices in Topczewski et al. (2017) spent time looking at all resonances in the spectra and did not discern the most critical areas of interest. Thus, organic chemistry students may require more practice using all spectral features together and models on expert approaches to interpreting the features all together. Students need to see how the features work together to be able to discern how to connect resonances with the structures and effectively perform checking procedures like experts and successful solvers ( Cartrette and Bodner, 2010 ). Worked examples that combine multiple spectral features could work well toward the end of the chapter, after the introduction of each spectral feature, and ideally after the students have had opportunities to use and master each spectral feature. Instructors could then point students towards additional models of expert-like solving through worked examples as well as problems to practice their understanding.
As we consider the framework on scaffolding from van de Pol et al. (2010) , this additive approach of spectral features within the worked examples and practice problems addresses the intent of scaffolding to reduce the degrees of freedom, allowing students to focus on and master interpretations with one spectral feature before introducing another degree of freedom in the form of another spectral feature. Building a student's fluency using spectral features from one to multiple moves from one degree of freedom to many. Increasing the degrees of freedom functions to fade support in scaffolded instruction. While we pointed out three textbooks that used an additive approach with the spectral features, our textbook analysis does not find any clear evidence of the textbooks helping establish mastery with additive scaffolding, where each spectral feature is first exercised alone and then in combination, in either the worked examples or practice problems. If we were to see evidence of scaffolding by adding in spectral features, we would expect the worked examples and practice problems within the chapter to block a number of problems on one spectral feature, before adding another spectral feature, before moving onto all four spectral features together. The Carey and Giuliano, and Smith textbooks both focus on mastering individual spectral features before moving onto additional spectral features. However, we note that there is not a clear signal for additive scaffolding in these books, as some spectral features are combined with others before covering the new feature on its own. A purposeful additive approach in scaffolding would cover one spectral feature on its own, then another on its own, and then combine the two features. A third spectral feature could then be introduced with practice problems of its own, and then either combined with the other two features or with one of the other features. Additive scaffolding in this sense then offers mastery of a single concept before adding another concept and increasing the degrees of freedom.
Support for instructional scaffolding by ordering spectral features
The lack of interleaving found in our sampled textbooks shines a light on an area in need of addressing. If textbooks utilized systematic blocking and interleaving it could be possible to mitigate the difficulties students have shown in problem solving with 1 H NMR spectra. Topczewski et al. (2017) showed that students spent time focusing on all resonances in the spectra, struggling to recognize or understand the connections needed to be made. Too much blocking could exacerbate issues such as these, where students could be led to believe that they must focus on and analyze all areas in the spectrum, using more of their working memory capacity in the process. Scaffolding could also aid in helping students see how the spectral data works together. Connor et al. (2019) found that students made assumptions that the N + 1 rule should hold true and that spectral data like typical chemical shift values should be absolute. In these cases, scaffolding and more blocking with each of the spectral features could help in picking out patterns within each of the spectral features alone, before having to use all spectral features together. Stowe and Cooper (2019) observed that students possessed procedural knowledge, but could not make well-supported arguments for the answers they found. In this situation, too much blocking could promote issues in knowing how to use all the information together.
Topczewski et al. (2017) also found in their study that students did not spend time connecting specific resonances with the structures. Here, interleaving could help students gain mastery in finding and connecting the relevant spectral support for a structure. Connor et al. (2019) also found that students used heuristics like rigidity and one-reason decision making, using only some or one of the spectral features to make their decisions. The use of such heuristics could be mitigated through interleaving, so students can understand how to use all spectral features together and see patterns across problems incorporating all spectral features. Since the students in Stowe and Cooper's (2019) study possessed adequate procedural knowledge and were able to arrive at the answers just fine, they needed help in using all the information. Interleaving could help students gain flexibility in using procedural knowledge and help students discern not only how to apply a strategy, but when to use that strategy ( Rohrer, 2012 ). Practice with interleaved problems would help students see how general rules in interpreting spectral features are not absolute and would provide students with opportunities to see the shortcomings of specific heuristics.
Our suggestion to block spectral features before interleaving contrasts with existing research conducted primarily in mathematics education. In math, substantial research supports the efficacy of interleaving in helping students develop robust problem-solving approaches ( Mayfield and Chase, 2002 ; Rohrer and Taylor, 2007 ; Rohrer et al. , 2014 ). Success in mathematics requires that students learn to choose appropriate strategies when solving problems. However, we believe blocking may be important in solving spectroscopy problems as students need to master interpretations with each spectral feature independently, before combining spectral features to solve a problem. Certainly, further research is warranted that explores the effects of interleaving and blocking on students’ ability to solve spectroscopy problems.
Conclusions
Although we focused on just one textbook chapter, we believe these results underscore a greater need to use the research from the learning and cognitive sciences and discipline-based education research to design textbooks. Much is known about problem solving and the importance of scaffolding and this research can inform textbook design, including the ordering and combination of worked examples and practice problems. A systematic approach to textbook design, including deliberate decisions about the order spectral features are introduced, using blocking and interleaving with practice problems, supports instructional scaffolding and by extension, student learning.
Limitations and future directions
Given the lack of worked examples throughout all four textbooks we analyzed, robust conclusions are limited. Examining the chapter narrative could provide further insight into the nuances of how the spectral features of 1 H NMR are described and taught. This research focuses on four baseline spectral features, but there are additional spectral features that become pertinent to chemists beyond the undergraduate level.
While we can use principles from the learning sciences to design textbooks, i.e. how we might order problems, further insights from students solving problems would help to refine and systematically shape textbook problems and worked examples. We know from Topczewski et al. (2017) that novice solvers pay attention to all four spectral features, but we do not know how students may struggle in discriminating between the spectral features and if they know when one spectral feature versus another provides them with the information necessary to solve for the structure of the problem. More research is needed that focuses on how students use and integrate the four spectral features of 1 H NMR spectroscopy presented here.
Some spectral features involve an understanding of others to be properly applied. For example, splitting and integration involve an understanding of proton equivalence. In our study, we only went as far as the prompts explicitly told students to solve for or reason with. The only problems that had an absence of specific features to solve for were the problems that asked for a whole structure, and those problems were coded for all four spectral features. Textbook analysis therefore contributes to this limitation, as we would not know if students are drawing on interrelated spectral features in that way or not. Stowe and Cooper's (2019) findings showed that as students displayed adequate procedural knowledge, they did not support their reasoning with spectral evidence. Likewise, the findings from Topczewski et al. (2017) , where novices did not connect spectral features with the provided structure answer choices, also shows that students are not drawing back to specific spectral features. We would not be sure if students were truly reasoning with the other features or not. Making such determinations turns into an examination of the problem-solving process and is therefore a facet of the individual human that cannot be determined from the textbook analysis itself.
Moreover, one spectral feature in a 1 H NMR spectrum can often be more critical than other features in determining the structure of the compound represented. On this level, blocking and interleaving could actually underly problems that would otherwise seem to assess all spectral features, such as the end-of-chapter problems for Brown et al. (2008) . It is possible that critical spectral features could be deliberately intermixed within a set of problems that, on the surface, cover all four spectral features. Future research should examine the nature of such critical spectral features and provide an intersection between the difficulties in solving students exhibit, as this involves aspects of the individual problem-solving process and characteristics of novice and expert solvers.
Implications
Implications for instruction, implications for research, conflicts of interest.
- Bodner G. M. and Domin D. S., (2000), Mental models: the role of representations in problem solving in chemistry, Univ. Chem. Educ. , 4 (1), 24–30.
- Brown W. H., Foote C. S., Iverson B. L. and Anslyn E. V., (2008), Organic Chemistry , 5th edn, Belmont: Brooks Cole, Cengage Learning.
- Bruice P. Y., (2004), Organic Chemistry , 4th edn, Upper Saddle River: Prentice Hall.
- Bruner J. S., (1974), From communication to language—a psychological perspective, Cognition , 3 (3), 255–287.
- Bruner J. S., (1983), Child's Talk - Learning to Use Language , 1st edn, New York: W.W. Norton.
- Carey F. A. and Giuliano R. M., (2011), Organic Chemistry , 8th edn, New York: McGraw-Hill.
- Cartrette D. P. and Bodner G. M., (2010), Non-mathematical problem solving in organic chemistry, J. Res. Sci. Teach. , 47 (6), 643–660.
- Chiappetta E. L., Fillman D. A. and Sethna G. H., (1991), A method to quantify major themes of scientific literacy in science textbooks, J. Res. Sci. Teach. , 28 (8), 713–725.
- Cooper M. M. and Stowe R. L., (2018), Chemistry education research—from personal empiricism to evidence, theory, and informed practice, Chem. Rev. , 118 (12), 6053–6087.
- Cooper M. M., Stowe R. L., Crandell O. M. and Klymkowsky M. W., (2019), Organic chemistry, life, the universe and everything (OCLUE): a transformed organic chemistry curriculum, J. Chem. Educ. , 96 (9), 1858–1872.
- Connor M. C. and Shultz G. V., (2018), Teaching assistants’ topic-specific pedagogical content knowledge in 1 H NMR spectroscopy, Chem. Educ. Res. Pract. , 19 (3), 653–669.
- Connor M. C., Finkenstaedt-Quinn S. A. and Shultz G. V., (2019), Constraints on organic chemistry students’ reasoning during IR and 1 H NMR spectral interpretation, Chem. Educ. Res. Pract. , 20 (3), 522–541.
- Cooper G. A. and Sweller J., (1987), Effects of schema acquisition and rule automation on mathematical problem-solving transfer, J. Educ. Psychol. , 79 (4), 347.
- Dávila K. and Talanquer V., (2010), Classifying end-of-chapter questions and problems for selected general chemistry textbooks used in the United States, J. Chem. Educ. , 87 (1), 97–101.
- Dickson S. V., Chard D. J. and Simmons D. C., (1993), An integrated reading/writing curriculum: a focus on scaffolding, LD Forum , 18 (4), 12–16.
- Domin D. S. and Bodner G. M., (2012), Using students’ representations constructed during problem solving to infer conceptual understanding, J. Chem. Educ. , 89 (7), 837–843.
- Gkitzia V., Salta K. and Tzougraki C., (2011), Development and application of suitable criteria for the evaluation of chemical representations in school textbooks, Chem. Educ. Res. Pract. , 12 (1), 5–14.
- Hmelo-Silver C. E., (2006), Design principles for scaffolding technology-based inquiry, in O’Donnell A. M., Hmelo-Silver C. M. and Erkens, G. (ed.), Collaborative Learning, Reasoning, and Technology , New York: Routledge, pp. 147–170.
- Jones Jr. M. and Fleming S. A., (2014), Organic Chemistry , 5th edn, New York: W. W. Norton & Company.
- Justi R. S. and Gilbert J. K., (2002), Modelling, teachers’ views on the nature of modelling, and implications for the education of modellers, Int. J. Sci. Educ. , 24 (4), 369–387.
- Kalyuga S., Chandler P., Tuovinen J. and Sweller J., (2001), When problem solving is superior to studying worked examples, J. Educ. Psychol. , 93 (3), 579.
- Knight B. A., (2015), Teachers’ use of textbooks in the digital age, Cogent Educ. , 2 (1), 1–10.
- Koppal M. and Caldwell A., (2004), Meeting the challenge of science literacy: Project 2061 efforts to improve science education, Cell Biol. Educ. , 3 (1), 28–30.
- Kornell, N. and Bjork R. A., (2008), Learning concepts and categories: is spacing the “enemy of induction”? Psychol. Sci. , 19 (6), 585–592.
- Kozma, R. B. and Russell J., (1997), Multimedia and understanding: expert and novice responses to different representations of chemical phenomena, J. Res. Sci. Teach. , 34 (9), 949–968.
- Lee C. S., McNeill N. J., Douglas E. P., Koro-Ljungberg M. E. and Therriault D. J., (2013), Indispensable resource? A phenomenological study of textbook use in engineering problem solving, J. Eng. Educ. , 102 (2), 268–288.
- Lin T., Hsu Y., Lin S., Changlai M., Yang K. and Lai T., (2012), A review of empirical evidence on scaffolding for science education, Int. J. Sci. Math. Educ. , 10 (2), 437–455.
- Maybin J., Mercer N. and Stierer B., (1992), Scaffolding learning in the classroom, in Norman K. (ed.), Thinking Voices: The Work of the National Oracy Project , London: Hodder and Stoughton, pp. 186–195.
- Mayfield K. H. and Chase P. N., (2002), The effects of cumulative practice on mathematics problem solving, J. Appl. Behav. Anal. , 35 (2), 105–123.
- McMurry J., (2012), Organic Chemistry , 8th edn, Belmont: Brooks Cole, Cengage Learning.
- McNeill K. L., Lizotte D. J., Krajcik J. and Marx R. W., (2006), Supporting students’ construction of scientific explanations by fading scaffolds in instructional materials, J. Learn. Sci. , 15 (2), 153–191.
- Mercer N. and Littleton K., (2007), Dialogue and the Development of Children's Thinking: A Sociocultural Approach , New York: Routledge.
- Mesa V. and Griffiths, B., (2012), Textbook mediation of teaching: an example from tertiary mathematics instructors, Educ. Stud. Math. , 79 (1), 85–107.
- Mikk J., (2000), Textbook: Research and Writing , New York: Peter Lang Publishing, Inc.
- Nyachwaya J. M. and Gillaspie M., (2016), Features of representations in general chemistry textbooks: a peek through the lens of the cognitive load theory, Chem. Educ. Res. Pract. , 17 (1), 58–71.
- Paas F. G. W. C., (1992), Training strategies for attaining transfer of problem solving skills in statistics: a cognitive-load approach, J. Educ. Psychol. , 84 (4), 429–434.
- Paas F. G. W. C. and van Merriënboer J. J. G., (1994), Variability of worked examples and transfer of geometrical problem-solving skills: a cognitive-load approach, J. Educ. Psychol. , 86 (1), 122–133.
- Paas F. G. W. C., van Merriënboer J. J. G. and Adam J. J., (2016), Measurement of cognitive load in instructional research, Percept. Mot. Skills , 79 (1), 419–430.
- Parker S. P., (1988), Spectroscopy Source Book , New York: McGraw-Hill.
- Pea R. D., (2004), The social and technological dimensions of scaffolding and related theoretical concepts for learning, education, and human activity, J. Learn. Sci. , 13 (3), 423–451.
- Puntambekar S. and Hubscher R., (2005), Tools for scaffolding students in a complex learning environment: what have we gained and what have we missed? Educ. Psychol. , 40 (1), 1–12.
- Pyburn D. T. and Pazicni S., (2014), Applying the multilevel framework of discourse comprehension to evaluate the text characteristics of general chemistry textbooks, J. Chem. Educ. , 91 (6), 778–783.
- Raker J. R. and Holme T. A., (2013), A historical analysis of the curriculum of organic chemistry using ACS exams as artifacts, J. Chem. Educ. , 90 (11), 1437–1442.
- Rohrer D., (2012), Interleaving helps students distinguish among similar concepts, Educ. Psychol. Rev. , 24 (3), 355–367.
- Rohrer D. and Taylor K. M., (2007), The shuffling of mathematics problems improves learning, Instr. Sci. , 35 (6), 481–498.
- Rohrer D., Dedrick R. F. and Burgess K., (2014), The benefit of interleaved mathematics practice is not limited to superficially similar kinds of problems, Psychon. Bull. Rev. , 21 (5), 1323–1330.
- Rosenshine B. and Meister C., (1992), The use of scaffolds for teaching higher-level cognitive strategies, Educ. Leadersh. , 49 (7), 26–33.
- Smith J. G., (2011), Organic Chemistry , 3rd edn, New York: McGraw-Hill.
- Solomons, T. W. G. and Fryhle C. B., (2008), Organic Chemistry , 9th edn, Hoboken: John Wiley.
- Stowe R. L. and Cooper M. M., (2019), Arguing from spectroscopic evidence, J. Chem. Educ. , 96 (10), 2072–2085.
- Tharp R. G. and Gallimore R., (1988), Rousing Minds to Life: Teaching, Learning, and Schooling in Social Context , New York: Cambridge University Press.
- Thomas N. C., (1991), The early history of spectroscopy, J. Chem. Educ. , 68 (8), 631.
- Topczewski J. J., Topczewski A. M., Tang H., Kendhammer L. K. and Pienta N. J., (2017), NMR spectra through the eyes of a student: eye tracking applied to NMR items, J. Chem. Educ. , 94 (1), 29–37.
- van de Pol J., Volman M. and Beishuizen J., (2010), Scaffolding in teacher–student interaction: a decade of research, Educ. Psychol. Rev. , 22 (3), 271–296.
- van Gerven P. W. M., Paas F. G. W. C., van Merriënboer J. J. G. and Schmidt H. G., (2002), Cognitive load theory and aging: effects of worked examples on training efficiency, Learn. Instr. , 12 (1), 87–105.
- Vlach H. A., Sandhofer C. M. and Kornell N., (2008), The spacing effect in children's memory and category induction, Cognition , 109 (1), 163–167.
- Vygotsky L. S., (1962), Thought and Language , Cambridge: MIT Press.
- Vygotsky L. S., (1978), Mind in Society: The Development of Higher Psychological Processes , Cambridge: Harvard University Press.
- Wade L. G., (2006), Organic Chemistry , 6th edn, Upper Saddle River: Prentice Hall.
- Wahlheim C. N., Dunlosky J. and Jacoby L. L., (2011), Spacing enhances the learning of natural concepts: an investigation of mechanisms, metacognition, and aging, Mem. Cogn. , 39 (5), 750–763.
- Weinberg A., Wiesner E., Benesh B. and Boester T., (2012), Undergraduate students’ self-reported use of mathematics textbooks, PRIMUS , 22 (2), 152–175.
- Wood D., Bruner J. S. and Ross G., (1976), The role of tutoring in problem solving, J. Child Psychol. Psychiatry , 17 (2), 89–100.
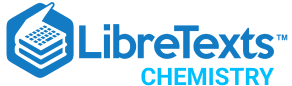
- school Campus Bookshelves
- menu_book Bookshelves
- perm_media Learning Objects
- login Login
- how_to_reg Request Instructor Account
- hub Instructor Commons
Margin Size
- Download Page (PDF)
- Download Full Book (PDF)
- Periodic Table
- Physics Constants
- Scientific Calculator
- Reference & Cite
- Tools expand_more
- Readability
selected template will load here
This action is not available.
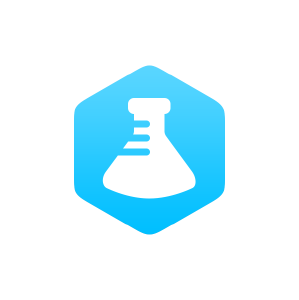
12.10.2 MS, IR and NMR Problems
- Last updated
- Save as PDF
- Page ID 42840
\( \newcommand{\vecs}[1]{\overset { \scriptstyle \rightharpoonup} {\mathbf{#1}} } \)
\( \newcommand{\vecd}[1]{\overset{-\!-\!\rightharpoonup}{\vphantom{a}\smash {#1}}} \)
\( \newcommand{\id}{\mathrm{id}}\) \( \newcommand{\Span}{\mathrm{span}}\)
( \newcommand{\kernel}{\mathrm{null}\,}\) \( \newcommand{\range}{\mathrm{range}\,}\)
\( \newcommand{\RealPart}{\mathrm{Re}}\) \( \newcommand{\ImaginaryPart}{\mathrm{Im}}\)
\( \newcommand{\Argument}{\mathrm{Arg}}\) \( \newcommand{\norm}[1]{\| #1 \|}\)
\( \newcommand{\inner}[2]{\langle #1, #2 \rangle}\)
\( \newcommand{\Span}{\mathrm{span}}\)
\( \newcommand{\id}{\mathrm{id}}\)
\( \newcommand{\kernel}{\mathrm{null}\,}\)
\( \newcommand{\range}{\mathrm{range}\,}\)
\( \newcommand{\RealPart}{\mathrm{Re}}\)
\( \newcommand{\ImaginaryPart}{\mathrm{Im}}\)
\( \newcommand{\Argument}{\mathrm{Arg}}\)
\( \newcommand{\norm}[1]{\| #1 \|}\)
\( \newcommand{\Span}{\mathrm{span}}\) \( \newcommand{\AA}{\unicode[.8,0]{x212B}}\)
\( \newcommand{\vectorA}[1]{\vec{#1}} % arrow\)
\( \newcommand{\vectorAt}[1]{\vec{\text{#1}}} % arrow\)
\( \newcommand{\vectorB}[1]{\overset { \scriptstyle \rightharpoonup} {\mathbf{#1}} } \)
\( \newcommand{\vectorC}[1]{\textbf{#1}} \)
\( \newcommand{\vectorD}[1]{\overrightarrow{#1}} \)
\( \newcommand{\vectorDt}[1]{\overrightarrow{\text{#1}}} \)
\( \newcommand{\vectE}[1]{\overset{-\!-\!\rightharpoonup}{\vphantom{a}\smash{\mathbf {#1}}}} \)
Solving molecular structures
Solving spectroscopy problems
Worked example
Putting it all together
Practice Problems
Using spectroscopy to determine structure
Really good practice
A workbook of unknowns
Spectroscopy worksheet
NMR/IR/MS practice problems
Spectroscopy practice problems
Practice problems IR/MS/NMR
Huge set of practice problems
Spectroscopy Practice exam and answers
** IR, MS and NMR practice exams
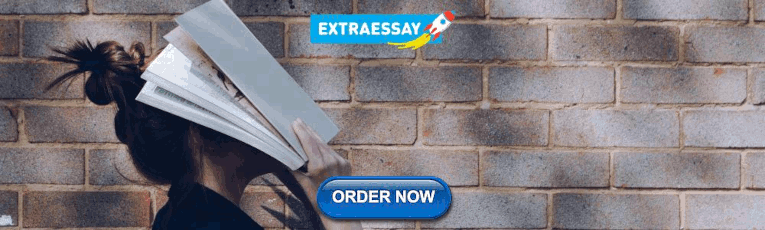
IMAGES
VIDEO
COMMENTS
NMR Practice Problems In the following examples, we will learn how to solve NMR practice problems step-by-step in over 100 min video solutions which is essential for organic structure determination.. The emphasis is on the 1 H proton NMR and most problems are based on understanding its key principles such as the number of NMR signals, integration, signal splitting (multiplicity), and, of ...
This video covers H-NMR Problems with detailed solutions. This is the problem solving video that we covered after the theory review of HNMR. If you have an...
Welcome to WebSpectra - This site was established to provide chemistry students with a library of spectroscopy problems. Interpretation of spectra is a technique that requires practice - this site provides 1 H NMR and 13 C NMR, DEPT, COSY and IR spectra of various compounds for students to interpret. Hopefully, these problems will provide a useful resource to better understand spectroscopy.
The following exercises are designed to help you become familiar with predicting the 1H NMR spectra of simple organic molecules. For each example you should find the number of signals you expect, where they should show on the scale (chemical shift), and what shape they should be (splitting patterns). Use the spectroscopy sheet to become ...
Proton NMR - Spectroscopy Peak Analysis Using C 3 H 7 Cl. H-NMR Example Matching the Molecule to the Graph. H-NMR Example (cont) Matching the Molecule to the Spectroscopy Graph. Slide Presentations. Interpretation of NMR. Interpreting NMR. Information for solving NMR. Web Pages *Interpreting NMR cartoon. Review of NMR problem solving. How to ...
This site was established to provide interested people in NMR with a library of NMR spectroscopy problems. Interpretation of spectra is a technique that requires pattern recognition and/or practice to order the chaos. This site provides one dimensional spectra of different nucleus, COSY, HSQC, HMBC and some less common spectra of various compounds to interprete.
This organic chemistry video provides a review of H NMR spectroscopy. It provides plenty of examples and multiple choice practice problems that you might en...
Solve unknown problems using 13 C and 1 H NMR spectra and molecular formula. Exercise \(\PageIndex{1}\) Which isomer of ortho, meta, or para xylene do you have based on the 13 C NMR spectrum?
Exercise 5.11.2 5.11. 2. Determine the structure for the unknown molecule with the molecular formula of C 5 H 10 O 2. 1 H NMR: The ratio of protons is 1:1:1:1:3:3. The peak at 9.5 ppm is a singlet. The peak at 2.41 ppm is sextet (J = 7 Hz). The peak at 1.72 ppm is a multiplet (J = 7Hz, 25 Hz). The peak at 1.53 ppm is a multiplet (J = 7 Hz, 25 ...
http://Leah4sci.com/NMR presents: Proton NMR Practice on Predicting Molecular Structure Using Formula + GraphNeed help with Orgo? Download my free guide '10 ...
Organic Chemistry 307 - Solving NMR Problems - H. D. Roth. Now we go to high field (right): there is a triplet (3 H) at 0.9 ppm; 3 H at high field is almost always a methyl group. The signal is a triplet (n + 1 = 3); therefore, the methyl group must have (n =) 2 1H neighbors; that must be a CH2group. The CH2 signal is a quartet (n + 1 = 4 ...
Topic Review on " NMR and Problem Solving ": NMR Background. Method of structure determination based upon the relative positions of hydrogens and carbons in the spectra. Only nuclei with an odd number of neutrons or an odd number of protons can give rise to an NMR signal. The most common nuclei are 1H and 13C.
H NMR Table Practice Problems. Enumerate the differences between the IR and 1 H NMR spectra of butyramide and those of N, N -diethylbutyramide. The methyl-H highlighted in the isopentane structure appears at 0.87 ppm, while that of 2-methylbut-2-ene appears at 1.56 ppm. Rationalize this observation.
H two is the CH. Okay, so then after that, what I'm going to get is h three, okay, Because H three is on a triple bond. And remember that triple bonds results a little bit lower than a flooring would result. Then we would get is a judge. Four, because if you notice h four is actually a Well, okay, so this would be an example of Z c. H.
37 PRACTICE PROBLEM. One Strong and another medium IR absorption at 1650 cm −1 and 1700 cm −1 were observed for an unknown compound with molecular formula C 3 H 3 BrO. The proton NMR spectrum of the compound shows two doublets at 6.7 ppm and 7.3 ppm, respectively, with J = 14 Hz and one singlet at 9.7 ppm. Predict the structure consistent ...
Solve unknown problems using a variety of spectra and the molecular formula. Exercise \(\PageIndex{1}\) ... The full 1 H NMR spectrum in D 2 O: An expansion: ... HMBC: Answer . Example \(\PageIndex{3}\) Propose a structure using the spectral data below for C 10 H 14 O. Note: You may need to check for solvent peaks. This sample was dissolved in ...
In this video, we will go over the strategies for solving NMR problems step by step. This practice problem involves determination of Hydrogen Deficiency Inde...
The description is a bit long (….so hold on!), but once you get it, you can just use the algorithm to solve your NMR problems. Here are some reference values and a couple of proton NMR spectra: Proton NMR Reference Values (cem.msu.edu) (mhhe.com) (process-nmr.com) (1H NMR of Taxol; unknown source) (Our example 1H NMR spectra for this post ...
The proton and carbon NMR spectra of a compound with the formula C 5 H 9 Br are shown below. The DEPT experimental results are also provided in the table. Purpose a plausible structure based on the data provided. I went over the steps for solving NMR problems with lots of examples which you can find here but for now let's quickly apply those ...
Additional NMR Examples. For each molecule, predict the number of signals in the 1 H-NMR and the 13 C-NMR spectra (do not count split peaks - eg. a quartet counts as only one signal). Assume that diastereotopic groups are non-equivalent. P5.2: For each of the 20 common amino acids, predict the number of signals in the proton-decoupled 13 C-NMR spectrum. P5.3: Calculate the chemical shift value ...
Worked examples and practice problems emerged from the analysis as primary resources existent in all the textbooks in supporting the learning of problem solving with 1 H NMR spectroscopy, as they offered opportunities to practice problem solving with the spectral features. A worked example was classified as a problem when it contained both an ...
Practice Problems. Multiple choice quiz. Multiple choice problems. Self-Assessment problems. On-line quiz. Great, Great GREAT Practice Set. NMR practice set. Key concepts of nmr with practice problems. NMR problems with answers. Good NMR practice problems. Multiple Choice NMR questions. Practice NMR problems. NMR quiz with answers
Solving molecular structures. Tutorial. Solving spectroscopy problems. Worked example. Putting it all together. Practice Problems. Using spectroscopy to determine structure. Really good practice. A workbook of unknowns. Spectroscopy worksheet. NMR/IR/MS practice problems. Spectroscopy practice problems. Practice problems IR/MS/NMR. Huge set of ...