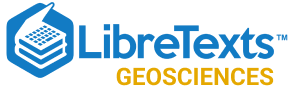
- school Campus Bookshelves
- menu_book Bookshelves
- perm_media Learning Objects
- login Login
- how_to_reg Request Instructor Account
- hub Instructor Commons
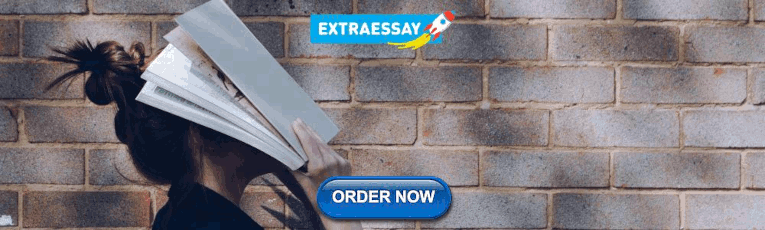
Margin Size
- Download Page (PDF)
- Download Full Book (PDF)
- Periodic Table
- Physics Constants
- Scientific Calculator
- Reference & Cite
- Tools expand_more
- Readability
selected template will load here
This action is not available.
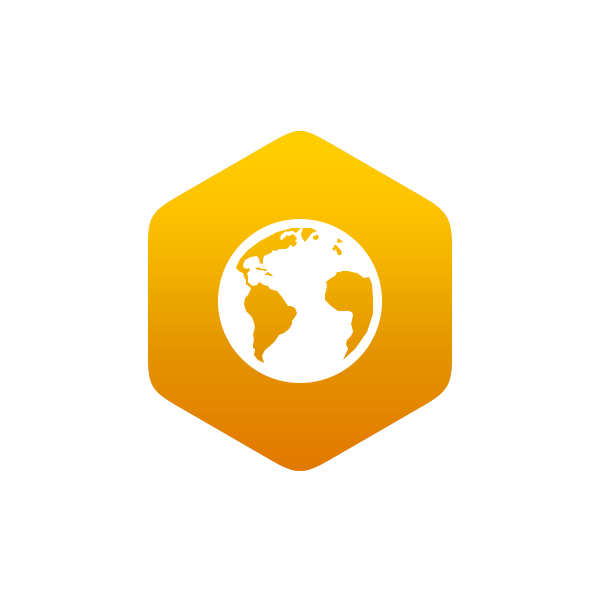
7.6: Effects of Volcanic Eruptions on Humans and on Earth Systems
- Last updated
- Save as PDF
- Page ID 25536
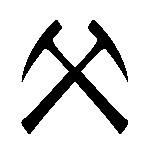
- Steven Earle
- Vancover Island University via BCCampus
\( \newcommand{\vecs}[1]{\overset { \scriptstyle \rightharpoonup} {\mathbf{#1}} } \)
\( \newcommand{\vecd}[1]{\overset{-\!-\!\rightharpoonup}{\vphantom{a}\smash {#1}}} \)
\( \newcommand{\id}{\mathrm{id}}\) \( \newcommand{\Span}{\mathrm{span}}\)
( \newcommand{\kernel}{\mathrm{null}\,}\) \( \newcommand{\range}{\mathrm{range}\,}\)
\( \newcommand{\RealPart}{\mathrm{Re}}\) \( \newcommand{\ImaginaryPart}{\mathrm{Im}}\)
\( \newcommand{\Argument}{\mathrm{Arg}}\) \( \newcommand{\norm}[1]{\| #1 \|}\)
\( \newcommand{\inner}[2]{\langle #1, #2 \rangle}\)
\( \newcommand{\Span}{\mathrm{span}}\)
\( \newcommand{\id}{\mathrm{id}}\)
\( \newcommand{\kernel}{\mathrm{null}\,}\)
\( \newcommand{\range}{\mathrm{range}\,}\)
\( \newcommand{\RealPart}{\mathrm{Re}}\)
\( \newcommand{\ImaginaryPart}{\mathrm{Im}}\)
\( \newcommand{\Argument}{\mathrm{Arg}}\)
\( \newcommand{\norm}[1]{\| #1 \|}\)
\( \newcommand{\Span}{\mathrm{span}}\) \( \newcommand{\AA}{\unicode[.8,0]{x212B}}\)
\( \newcommand{\vectorA}[1]{\vec{#1}} % arrow\)
\( \newcommand{\vectorAt}[1]{\vec{\text{#1}}} % arrow\)
\( \newcommand{\vectorB}[1]{\overset { \scriptstyle \rightharpoonup} {\mathbf{#1}} } \)
\( \newcommand{\vectorC}[1]{\textbf{#1}} \)
\( \newcommand{\vectorD}[1]{\overrightarrow{#1}} \)
\( \newcommand{\vectorDt}[1]{\overrightarrow{\text{#1}}} \)
\( \newcommand{\vectE}[1]{\overset{-\!-\!\rightharpoonup}{\vphantom{a}\smash{\mathbf {#1}}}} \)
Humans have a love-hate relationship with volcanoes. For many reasons humans are attracted to areas with active volcanism, but for several others that we’ve already discussed, they would be wise to stay away.
The key reason that humans like living around potentially active volcanoes is that the soil tends to be fertile, and thus there is the potential to grow enough food to live. For example, some parts of the area around Mt. Merapi in Indonesia (Figure 7.0.1) can support subsistence populations of 8 to 10 people per hectare. [1] In comparison, the typical farm in the United States can feed just under 1 person per hectare ( US Farm Bureau ).
Volcanic soil is good for a number of reasons. One is that volcanic ash and rock fragments are rich in volcanic glass and under weathering conditions glass breaks down quickly to clay minerals so that productive soil can form within 200 to 300 years in favorable climates. [2] Another is that the clays that form from volcanic parent materials are effective at holding onto nutrients such as phosphorous. A third is that volcanic lava or tephra are typically quite rich in some important plant nutrients, such as magnesium and sulphur. Volcanic regions all over the world are know for their fertile soils. Some examples, apart from Indonesia, include the volcanic areas in Italy, much of northern New Zealand, Japan, Hawaii, parts of Africa, and much of the Caribbean.
Volcanoes are also valued for their scenic beauty and recreational opportunities. An example is the Mt. Garibaldi area of southwestern British Columbia (Figure 7.6.1), but there are hundreds of other scenic volcanoes around the world, some of which are immense tourist and hiker attractions (Figure 7.6.2). Many volcanoes are also venues for a wide range of winter sports, and for hot springs, spas and mudbaths. Volcanic regions are also an excellent source of geothermal heat for both electricity and district heating, and of hydroelectric energy from streams.
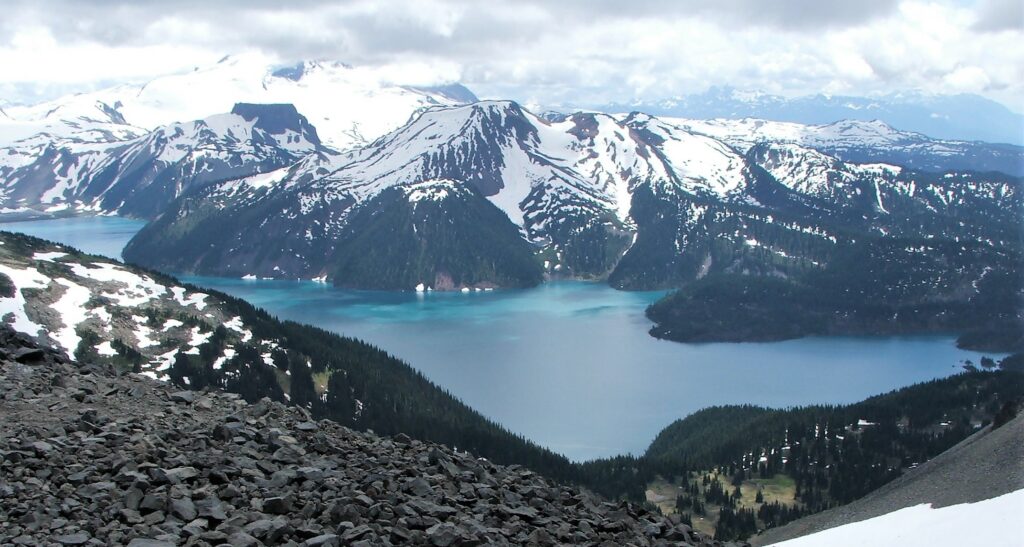
Many volcanoes are also venues for a wide range of winter sports, and for hot springs, spas and mudbaths. Volcanic regions are also an excellent source of geothermal heat for both electricity and district heating, and of hydroelectric energy from streams. Figure 7.6.3 provides an overview of some of the ways that humans interact with volcanoes, and some of the risks associated with living nearby.
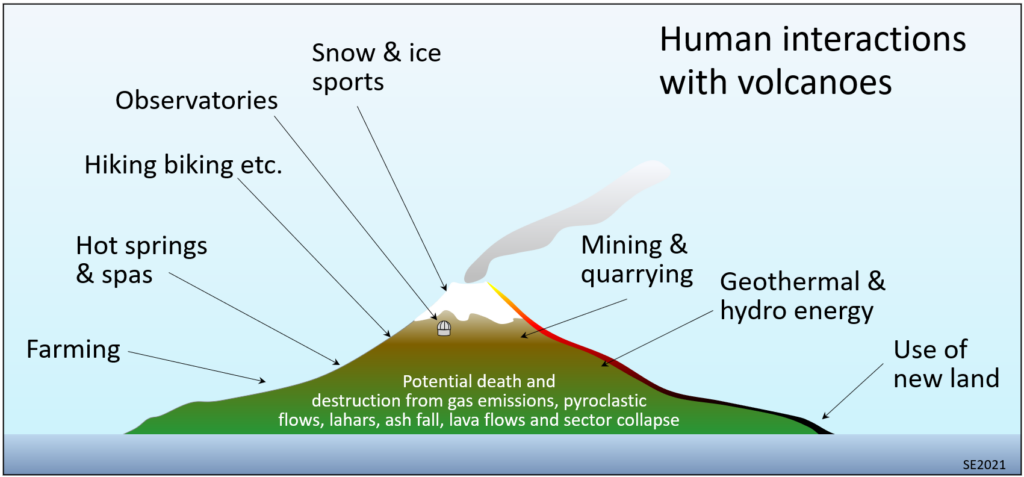
Volcanism and Earth Systems
As already noted in Chapter 1 and Chapter 3 , volcanic eruptions contribute to the Earth’s systems in important ways. For starters, it is widely believed that the water in the Earth’s oceans is at least partly derived from volcanism, and the Earth would not have much in the way of systems without water.
Some of the key roles of volcanic eruptions in Earth systems are as follows:
- Cycling solids (mostly silicates) from depth in the mantle and the crust to surface,
- Cycling volatiles (water and gases) from depth, and thereby influencing organisms and the climate,
- Ejecting both solids and volatiles high into the atmosphere,
- Cycling thermal energy from depth,
- Creating solid surfaces (e.g., islands) that will be colonized by organisms, and
- Creating sloped surfaces (mountains) that influence weather and climate patterns, and will be eroded and weathered.
All of these products subsequently contribute to other Earth system processes in myriad ways.
Media Attributions
- Figure 7.6.1 Photo by Isaac Earle, used with permission, CC BY 4.0
- Figure 7.6.2 Mt. Fuji Summit by Derek Mawhinney, public domain image via Wikimedia Commons, https://commons.wikimedia.org/wiki/F...uji_Summit.jpg
- Figure 7.6.3 Steven Earle, CC BY 4.0
- Dahlgren, R., Saigusa, M., & Ugolini, F. (2004). The nature, properties and management of volcanic soils. Advances in Agronom y, 82 , 114-183. https://doi.org/10.1016/S0065-2113(03)82003-5 ↵
- (Dahlgern et al., 2004) ↵
- Engineering Geology
- Field Methods
- Historical Geology
- Hydrogeology
- Earth Interior
- Geologic Time Scale
- Plate Tectonics
- Geologic Lists
- Geological Wonders
- Igneous Rocks
- Extrusive Igneous Rocks
- Intrusive Igneous Rocks
- Metamorphic Rocks
- Foliated Metamorphic Rocks
- Sedimentary Rocks
- Metamorphic rocks
- Borate minerals
- Carbonates Minerals
- Halide Minerals
- Native Mineral
- Physical Properties
- Optical Properties
- Earthquakes
- Volcanic Eruption
- Privacy Policy
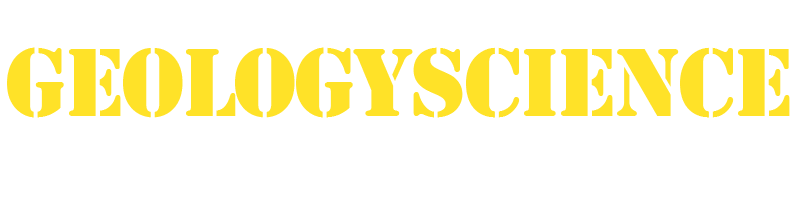
- Volcanology
Causes of Volcanic Eruptions
Volcanic eruptions are awe-inspiring natural phenomena that have fascinated and perplexed humanity throughout history. These explosive events, driven by the Earth’s internal processes, shape landscapes and impact ecosystems in profound ways. Understanding the causes of volcanic eruptions is crucial for both scientific exploration and mitigating potential hazards associated with volcanic activity.

A volcanic eruption refers to the sudden release of magma, ash, and gases from the Earth’s interior through vents or fissures on the surface. This dynamic process can result in the formation of new landforms , such as mountains, craters, and lava plateaus. Volcanic eruptions vary widely in scale, ranging from minor effusive flows to catastrophic explosive events that can alter global climate patterns.
Significance of Studying Volcanic Eruptions:
Studying volcanic eruptions holds immense significance for several reasons. First and foremost, it provides crucial insights into the Earth’s internal dynamics, helping scientists unravel the mysteries of our planet’s composition and evolution. Additionally, understanding volcanic activity is essential for assessing and managing potential risks associated with eruptions, such as lava flows, pyroclastic flows, and ashfall, which can pose threats to human life, infrastructure, and agriculture.
Furthermore, volcanic eruptions play a pivotal role in shaping the Earth’s surface and influencing ecosystems. The deposition of volcanic materials enriches soils, fostering unique biodiversity in volcanic regions. The gases released during eruptions can also contribute to atmospheric processes, influencing climate patterns on both local and global scales.
Types of Volcanic Activity:
Volcanic activity manifests in various forms, each with distinct characteristics and consequences. The two primary types of volcanic eruptions are effusive and explosive.
- Effusive Eruptions: These eruptions involve the relatively gentle release of magma, often resulting in the flow of lava. Lava may emerge through fissures or vents, forming shield volcanoes or lava plateaus. Effusive eruptions are typically associated with low-viscosity magma, allowing it to flow more freely.
- Explosive Eruptions: Characterized by violent and sudden releases of pressure, explosive eruptions eject ash, gases, and volcanic rocks into the atmosphere. This type of eruption can result in the formation of composite volcanoes, calderas, and pyroclastic flows. Explosive eruptions are often linked to high-viscosity magma, which traps gases and builds up pressure beneath the Earth’s surface.
In summary, understanding the causes and mechanisms behind volcanic eruptions is crucial for scientific inquiry, risk assessment, and environmental management. By delving into the intricacies of volcanic activity, researchers can unravel the mysteries of our planet’s dynamic processes and develop strategies to mitigate the potential impact of volcanic events on human communities and the natural environment.
Earth’s Interior Structure
Magma formation, tectonic plate boundaries, volcanic hotspots, volcanic triggering mechanisms, historic volcanic eruption.

The Earth’s interior is composed of several distinct layers, each characterized by unique physical and compositional properties. These layers, from the outermost to the innermost, are the crust, mantle, outer core, and inner core. The study of the Earth’s interior structure is known as seismology, and it relies on the analysis of seismic waves generated by earthquakes to infer the properties of these layers.
- The Earth’s outermost layer is called the crust.
- It is relatively thin compared to the other layers, ranging from about 5 to 70 kilometers in thickness.
- The crust is divided into two types: continental crust, which forms the continents, and oceanic crust, which underlies the ocean basins.
- Composed primarily of solid rocks, the crust is rich in silicate minerals .
- Beneath the crust lies the mantle, extending to a depth of about 2,900 kilometers.
- The mantle is predominantly composed of solid rock, but it can exhibit semi-fluid behavior over geological timescales, allowing it to flow slowly.
- This layer experiences convection currents, driven by heat from the Earth’s interior. These currents play a crucial role in the movement of tectonic plates.
- Below the mantle is the outer core, extending from a depth of approximately 2,900 to 5,150 kilometers.
- The outer core is composed mainly of molten iron and nickel . The liquid state of these metals is inferred from the inability of shear waves (a type of seismic wave) to travel through it.
- The movement of molten iron and nickel in the outer core generates Earth’s magnetic field through a process called the geodynamo.
- The innermost layer of the Earth, extending from a depth of about 5,150 kilometers to the center at approximately 6,371 kilometers, is the inner core.
- Despite high temperatures, the inner core remains solid due to intense pressure.
- Composed mainly of iron and nickel, the inner core’s solid nature is inferred from the behavior of seismic waves.
The transitions between these layers are not sharp boundaries but rather gradual changes in temperature, pressure, and material properties. The Earth’s interior is a dynamic system with heat flows, convection currents, and other processes that contribute to the planet’s geological activity and surface features, such as earthquakes, volcanic eruptions, and the movement of tectonic plates. Seismological studies, in conjunction with other geological and geophysical methods , continue to enhance our understanding of the complexities of the Earth’s interior structure.

Magma formation is a process that occurs beneath the Earth’s surface, where rocks melt to create a molten mixture of minerals. This molten material, known as magma, is a key component in the formation of igneous rocks and is often associated with volcanic activity. The process of magma formation involves a combination of heat, pressure, and the composition of the Earth’s mantle.
Here are the primary factors and processes involved in magma formation:
- Heat is a fundamental factor in magma formation. As one descends deeper into the Earth, temperatures increase. The heat needed for magma formation comes from several sources, including the residual heat from the planet’s formation, radioactive decay of certain elements in the Earth’s mantle, and heat generated by the movement of molten material.
- Pressure also plays a role in magma formation. As rocks descend into the Earth’s interior, they encounter higher pressures. This pressure can suppress the melting of rocks, even at elevated temperatures. However, when rocks move to shallower depths or experience a decrease in pressure through processes like tectonic plate movement or mantle upwelling, they are more likely to melt.
- The composition of rocks is a critical factor in magma formation. Different minerals have different melting points. Rocks are composed of various minerals, and when the temperature exceeds the melting point of certain minerals within a rock, those minerals will begin to melt, contributing to the formation of magma. The composition of the magma depends on the minerals present in the original rocks.
- The presence of water also influences magma formation. Water can lower the melting point of rocks, making it easier for them to undergo partial melting. Water is often introduced into the mantle through subduction zones, where oceanic plates sink beneath continental plates, carrying water with them.
- Upwelling of magma from the mantle is another process that contributes to magma formation. Mantle plumes, which are hot, buoyant upwellings of material from deep within the Earth, can lead to the melting of rock and the generation of magma. This is thought to be a significant factor in the formation of hotspot volcanoes.
Once magma is formed, it can rise towards the Earth’s surface due to its lower density compared to the surrounding solid rock. The ascent of magma can lead to volcanic activity, where it may erupt onto the surface as lava, ash, and gases.
Understanding the processes of magma formation is crucial for comprehending volcanic activity and the Earth’s dynamic internal processes. Researchers use various methods, including laboratory experiments, field studies, and seismic observations, to investigate and model the conditions under which magma is generated within the Earth.

Tectonic plate boundaries play a fundamental role in the causes of volcanic eruptions. The Earth’s lithosphere is divided into several large plates that float on the semi-fluid asthenosphere beneath them. The interactions between these plates at their boundaries create conditions conducive to the formation and eruption of volcanoes. There are three main types of plate boundaries associated with volcanic activity: divergent boundaries, convergent boundaries, and transform boundaries.
- At divergent boundaries, tectonic plates move away from each other. As plates separate, magma from the mantle rises to fill the gap, creating new oceanic crust through a process known as seafloor spreading.
- The rising magma can breach the ocean floor, leading to the formation of underwater volcanoes and mid-ocean ridges. These volcanic eruptions are typically characterized by effusive lava flows.
- Convergent boundaries involve the collision or subduction of tectonic plates. When an oceanic plate collides with a continental plate, or when two continental plates converge, the denser oceanic plate is usually forced beneath the lighter continental plate in a process called subduction.
- As the subducting plate sinks into the mantle, it undergoes partial melting due to the increase in temperature and pressure. The melted rock (magma) rises through the overlying plate, leading to the formation of magma chambers beneath the Earth’s surface.
- The magma can eventually reach the surface, causing explosive volcanic eruptions. These eruptions are often associated with the formation of volcanic arcs and can be particularly violent due to the viscosity of the magma and the release of trapped gases.
- At transform boundaries, tectonic plates slide past each other horizontally. While transform boundaries are not typically associated with large volcanic mountain formations, they can contribute to the formation of volcanic activity under certain circumstances.
- Frictional forces at transform boundaries can generate heat, and localized melting may occur, leading to the formation of magma. Volcanic activity at transform boundaries is usually less intense compared to convergent boundaries.
In summary, the movement and interactions of tectonic plates at plate boundaries are central to the causes of volcanic eruptions. Whether plates are diverging, converging, or sliding past each other, the associated geological processes create conditions conducive to magma formation and the release of volcanic activity. The diverse nature of volcanic eruptions around the world can be attributed to the dynamic interactions at these tectonic plate boundaries.

Volcanic hotspots are areas on the Earth’s surface where volcanic activity is unusually high, often resulting in the formation of volcanic features such as magma plumes, basaltic lava flows, and volcanic islands. Unlike volcanic activity at tectonic plate boundaries, hotspots are thought to be stationary relative to the moving tectonic plates. The exact mechanism behind the formation of hotspots is still a subject of scientific investigation, but they are believed to be associated with mantle plumes—hot, buoyant upwellings of molten rock originating from deep within the Earth.
Key characteristics and features of volcanic hotspots include:
- The prevailing theory suggests that volcanic hotspots are caused by mantle plumes—long, narrow columns of hot rock that rise from the boundary between the Earth’s core and mantle. As these plumes reach the mantle’s upper boundary, they can induce melting, creating magma chambers.
- Unlike most volcanic activity associated with tectonic plate boundaries, hotspots are often considered to be relatively stationary. This leads to a chain of volcanic activity, with older volcanic structures becoming progressively younger as they move away from the hotspot.
- Hotspots can generate volcanic chains or trails of islands, seamounts, and volcanic features as tectonic plates move over them. The Hawaiian Islands are a classic example of a hotspot volcanic chain.
- Hotspot activity beneath oceanic crust can result in the formation of volcanic islands. As magma rises to the surface, it can build up layers of solidified lava, forming islands. Over time, as the tectonic plate moves, a chain of islands is created.
- Hotspot volcanic chains often exhibit a gradient of geological ages, with the youngest volcanic structures located above the current position of the hotspot. The older volcanic islands or seamounts in the chain are progressively eroded or subside below sea level.
- The Hawaiian-Emperor seamount chain is a well-known example of a hotspot track. The Yellowstone hotspot, located beneath Yellowstone National Park in the United States, is another example that has resulted in significant volcanic activity.
It’s important to note that the exact nature and origin of mantle plumes and hotspots are still areas of active research, and scientific understanding of these phenomena continues to evolve. Hotspots provide valuable insights into the dynamics of the Earth’s mantle and contribute to the geological diversity observed on the planet’s surface.

Volcanic eruptions can be triggered by various mechanisms, and while the exact causes can be complex and multifaceted, here are some key triggering mechanisms:
- Subduction Zones: In convergent plate boundaries, where one tectonic plate is forced beneath another (subduction), intense heat and pressure can cause the subducting plate to melt, leading to the formation of magma. This magma can then rise to the surface, triggering volcanic eruptions.
- Rifting: At divergent plate boundaries, where tectonic plates move apart, magma from the mantle can intrude into the gap, leading to the creation of new crust. This process, known as rifting, is associated with volcanic activity, particularly along mid-ocean ridges.
- Mantle Plumes: Hot, buoyant upwellings of molten rock from the Earth’s mantle, known as mantle plumes, can lead to the formation of hotspots. As the plume reaches the crust, it can induce melting, creating magma chambers that feed volcanic activity. The movement of tectonic plates over hotspots can create chains of volcanic islands.
- Geothermal Energy Extraction: Human activities, such as geothermal energy extraction, can sometimes induce volcanic activity. The extraction of fluids from geothermal reservoirs can alter the pressure conditions in the subsurface and potentially trigger volcanic eruptions.
- Dome Instability: Volcanic domes are formed by the extrusion of lava with high viscosity. The weight of the lava on the dome can lead to instability, causing partial or complete collapse. The collapse can release trapped gas and magma pressure, leading to explosive eruptions.
- Tectonic Earthquakes: Earthquakes, especially those associated with tectonic activity, can sometimes trigger volcanic eruptions. The seismic activity can cause changes in pressure and create fractures in the Earth’s crust, facilitating the ascent of magma.
- Gas Overpressure: The accumulation of gas within a magma chamber can lead to increased pressure. If the gas pressure surpasses the confining strength of the rocks, it can trigger an explosive eruption.
- Meteorite Impact: Although rare, a large meteorite impact on the Earth’s surface has the potential to generate enough heat and pressure to melt rocks and initiate volcanic activity.
- Glacial Retreat: Changes in ice volume due to glacial retreat can influence volcanic activity. The removal of the weight of glacial ice may lead to decompression melting in the underlying mantle, contributing to volcanic eruptions.
Understanding these triggering mechanisms is essential for assessing volcanic hazards and mitigating potential risks associated with eruptions. Volcanic monitoring systems, geological studies, and advances in seismology contribute to ongoing efforts to comprehend and predict volcanic activity.

1. Mount Vesuvius, AD 79:
- Event: The eruption of Mount Vesuvius in AD 79 is one of the most infamous volcanic events in history. It buried the Roman cities of Pompeii and Herculaneum under a thick layer of ash and pumice .
- Causes: Mount Vesuvius is located near the convergent boundary of the African and Eurasian tectonic plates. The eruption was a result of the subduction of the African plate beneath the Eurasian plate, leading to the accumulation of magma beneath the surface.
- Lessons Learned: The catastrophic impact of the Vesuvius eruption underscores the importance of understanding the geological setting of volcanic regions. It also emphasizes the need for effective evacuation plans and early warning systems for populations living near active volcanoes.
2. Krakatoa, 1883:
- Event: The eruption of Krakatoa in 1883, located between the islands of Java and Sumatra, resulted in one of the most powerful volcanic explosions in recorded history. The eruption led to tsunamis, global climate effects, and the collapse of the island.
- Causes: Krakatoa’s eruption was caused by the collapse of the volcanic island due to a combination of magma chamber overpressure and tectonic activity in the Sunda Strait.
- Lessons Learned: Krakatoa highlighted the far-reaching consequences of volcanic eruptions, including tsunamis and atmospheric effects. It emphasized the importance of international cooperation in monitoring and mitigating global impacts.
3. Mount St. Helens , 1980:
- Event: The eruption of Mount St. Helens in 1980 in the state of Washington, USA, was a highly destructive event. The eruption resulted in the lateral collapse of the volcano’s north flank and the release of a massive debris avalanche.
- Causes: Mount St. Helens is located at a convergent plate boundary where the Juan de Fuca plate subducts beneath the North American plate. The eruption was triggered by the release of pressure from the magma chamber and the collapse of the unstable north flank.
- Lessons Learned: The eruption highlighted the need for improved monitoring of volcanic precursors, such as ground deformation and gas emissions. It also emphasized the importance of land-use planning to mitigate the impact on surrounding communities.
4. Pinatubo, 1991:
- Event: The eruption of Mount Pinatubo in the Philippines in 1991 was one of the largest volcanic eruptions of the 20th century. It had significant global climatic impacts.
- Causes: The eruption was triggered by the injection of magma into the volcano’s chamber, leading to increased pressure. The climactic eruption released a large volume of ash and sulfur dioxide into the stratosphere.
- Lessons Learned: Pinatubo highlighted the potential for volcanic eruptions to influence global climate. The monitoring and study of volcanic gas emissions gained increased importance in assessing potential impacts on the atmosphere.
5. Eyjafjallajökull, 2010:
- Event: The eruption of Eyjafjallajökull in Iceland in 2010 disrupted air travel across Europe due to the release of volcanic ash into the atmosphere.
- Causes: The eruption was caused by the interaction of magma with ice, leading to explosive activity. The ash cloud created aviation hazards and prompted widespread airspace closures.
- Lessons Learned: The Eyjafjallajökull eruption underscored the vulnerability of air travel to volcanic ash. It highlighted the need for improved communication and coordination between volcanic monitoring agencies and aviation authorities.
Implications for Future Monitoring:
- Advances in satellite technology, ground-based monitoring systems, and improved understanding of volcanic precursors are crucial for early detection and warning.
- International collaboration and information-sharing are essential for managing the impact of volcanic events, especially those with global consequences.
- Public awareness and education about volcanic risks and evacuation plans are key components of preparedness.
- Ongoing research into volcanic processes, including gas emissions and magma behavior, contributes to better forecasting and risk assessment.
These case studies demonstrate the diverse causes and impacts of volcanic eruptions and highlight the ongoing efforts to learn from past events for more effective monitoring and mitigation in the future.
In conclusion, the causes of volcanic eruptions are multifaceted and often stem from dynamic processes within the Earth’s interior. The interplay of geological forces at tectonic plate boundaries and other volcanic features such as hotspots contributes to the diverse and spectacular volcanic activity observed around the world.
Tectonic plate interactions, including subduction, divergence, and lateral sliding, play a pivotal role in triggering volcanic events. Subduction zones, where one plate descends beneath another, can lead to the melting of rock and the formation of magma. Divergent boundaries, where plates move apart, allow magma to rise from the mantle, creating new crust. Transform boundaries, where plates slide past each other, can generate heat and localized melting.
Mantle plumes and hotspots provide another mechanism for magma generation. These upwellings of hot rock from the Earth’s mantle can create stationary points of intense volcanic activity, forming volcanic island chains and contributing to the geological diversity of the planet.
Human activities, such as geothermal energy extraction, can also influence volcanic activity, albeit on a smaller scale. Additionally, external triggers like meteorite impacts and climate-related factors, such as glacial retreat, may contribute to volcanic events.
Historic volcanic eruptions serve as valuable case studies, offering insights into the complex causes and far-reaching consequences of such events. Lessons learned from events like the eruption of Mount Vesuvius, Krakatoa, Mount St. Helens, Pinatubo, and Eyjafjallajökull underscore the importance of understanding volcanic hazards, implementing effective monitoring systems, and developing strategies for risk mitigation.
Advancements in seismology, satellite technology, and the study of volcanic gas emissions contribute to ongoing efforts to monitor and predict volcanic activity. Public awareness, education, and international collaboration are essential components of preparedness and response to volcanic events.
In navigating the intricate processes that lead to volcanic eruptions, the scientific community continues to deepen its understanding, striving for improved forecasting, risk assessment, and the development of strategies to safeguard communities living in volcanic regions. As we move forward, the pursuit of knowledge about the Earth’s dynamic interior remains crucial for enhancing our ability to coexist with the natural forces that shape our planet.
RELATED ARTICLES MORE FROM AUTHOR
Volcano anatomy, types of volcanic eruptions, decade volcanoes, hawaii volcanoes national park, usa, 10 most powerful super volcanoes, mount st. helens volcano, washington, usa, recent posts, coober pedy opal, persian turquoise.

Volcanic Eruptions and Their Repose, Unrest, Precursors, and Timing (2017)
Chapter: 1 introduction, 1 introduction.
Volcanoes are a key part of the Earth system. Most of Earth’s atmosphere, water, and crust were delivered by volcanoes, and volcanoes continue to recycle earth materials. Volcanic eruptions are common. More than a dozen are usually erupting at any time somewhere on Earth, and close to 100 erupt in any year ( Loughlin et al., 2015 ).
Volcano landforms and eruptive behavior are diverse, reflecting the large number and complexity of interacting processes that govern the generation, storage, ascent, and eruption of magmas. Eruptions are influenced by the tectonic setting, the properties of Earth’s crust, and the history of the volcano. Yet, despite the great variability in the ways volcanoes erupt, eruptions are all governed by a common set of physical and chemical processes. Understanding how volcanoes form, how they erupt, and their consequences requires an understanding of the processes that cause rocks to melt and change composition, how magma is stored in the crust and then rises to the surface, and the interaction of magma with its surroundings. Our understanding of how volcanoes work and their consequences is also shared with the millions of people who visit U.S. volcano national parks each year.
Volcanoes have enormous destructive power. Eruptions can change weather patterns, disrupt climate, and cause widespread human suffering and, in the past, mass extinctions. Globally, volcanic eruptions caused about 80,000 deaths during the 20th century ( Sigurdsson et al., 2015 ). Even modest eruptions, such as the 2010 Eyjafjallajökull eruption in Iceland, have multibillion-dollar global impacts through disruption of air traffic. The 2014 steam explosion at Mount Ontake, Japan, killed 57 people without any magma reaching the surface. Many volcanoes in the United States have the potential for much larger eruptions, such as the 1912 eruption of Katmai, Alaska, the largest volcanic eruption of the 20th century ( Hildreth and Fierstein, 2012 ). The 2008 eruption of the unmonitored Kasatochi volcano, Alaska, distributed volcanic gases over most of the continental United States within a week ( Figure 1.1 ).
Finally, volcanoes are important economically. Volcanic heat provides low-carbon geothermal energy. U.S. generation of geothermal energy accounts for nearly one-quarter of the global capacity ( Bertani, 2015 ). In addition, volcanoes act as magmatic and hydrothermal distilleries that create ore deposits, including gold and copper ores.
Moderate to large volcanic eruptions are infrequent yet high-consequence events. The impact of the largest possible eruption, similar to the super-eruptions at Yellowstone, Wyoming; Long Valley, California; or Valles Caldera, New Mexico, would exceed that of any other terrestrial natural event. Volcanoes pose the greatest natural hazard over time scales of several decades and longer, and at longer time scales they have the potential for global catastrophe ( Figure 1.2 ). While
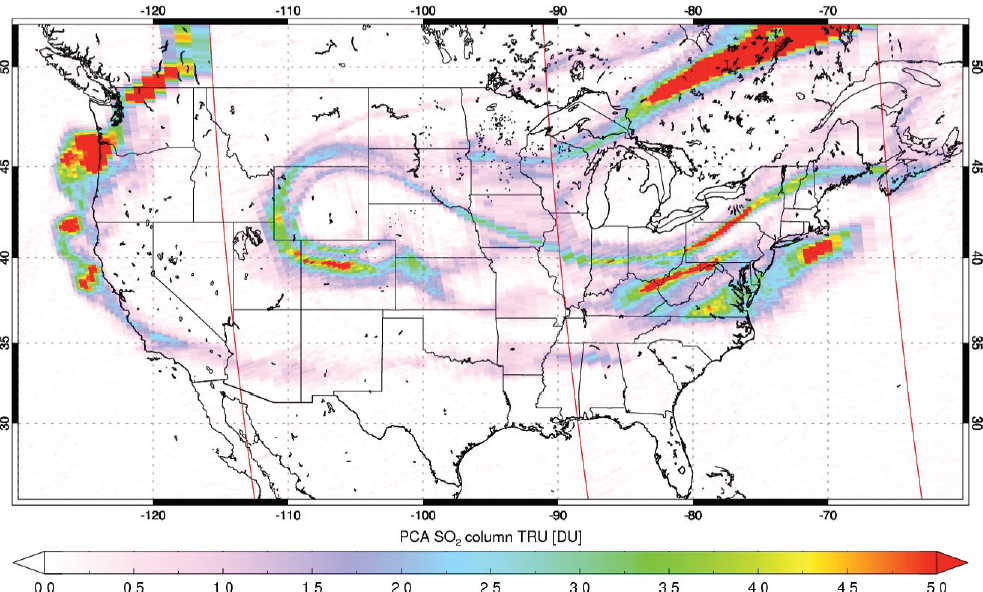
the continental United States has not suffered a fatal eruption since 1980 at Mount St. Helens, the threat has only increased as more people move into volcanic areas.
Volcanic eruptions evolve over very different temporal and spatial scales than most other natural hazards ( Figure 1.3 ). In particular, many eruptions are preceded by signs of unrest that can serve as warnings, and an eruption itself often persists for an extended period of time. For example, the eruption of Kilauea Volcano in Hawaii has continued since 1983. We also know the locations of many volcanoes and, hence, where most eruptions will occur. For these reasons, the impacts of at least some types of volcanic eruptions should be easier to mitigate than other natural hazards.
Anticipating the largest volcanic eruptions is possible. Magma must rise to Earth’s surface and this movement is usually accompanied by precursors—changes in seismic, deformation, and geochemical signals that can be recorded by ground-based and space-borne instruments. However, depending on the monitoring infrastructure, precursors may present themselves over time scales that range from a few hours (e.g., 2002 Reventador, Ecuador, and 2015 Calbuco, Chile) to decades before eruption (e.g., 1994 Rabaul, Papua New Guinea). Moreover, not all signals of volcanic unrest are immediate precursors to surface eruptions (e.g., currently Long Valley, California, and Campi Flegrei, Italy).
Probabilistic forecasts account for this uncertainty using all potential eruption scenarios and all relevant data. An important consideration is that the historical record is short and biased. The instrumented record is even shorter and, for most volcanoes, spans only the last few decades—a miniscule fraction of their lifetime. Knowledge can be extended qualitatively using field studies of volcanic deposits, historical accounts, and proxy data, such as ice and marine sediment cores and speleothem (cave) records. Yet, these too are biased because they commonly do not record small to moderate eruptions.
Understanding volcanic eruptions requires contributions from a wide range of disciplines and approaches. Geologic studies play a critical role in reconstructing the past eruption history of volcanoes,
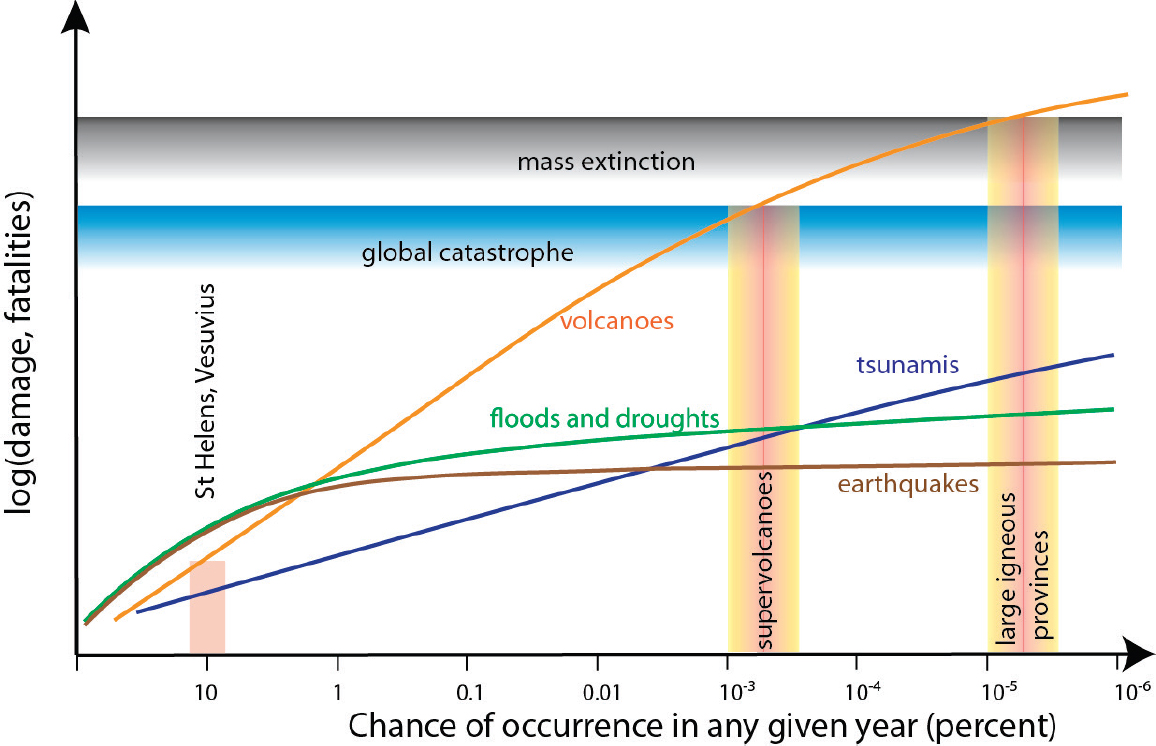
especially of the largest events, and in regions with no historical or directly observed eruptions. Geochemical and geophysical techniques are used to study volcano processes at scales ranging from crystals to plumes of volcanic ash. Models reveal essential processes that control volcanic eruptions, and guide data collection. Monitoring provides a wealth of information about the life cycle of volcanoes and vital clues about what kind of eruption is likely and when it may occur.
1.1 OVERVIEW OF THIS REPORT
At the request of managers at the National Aeronautics and Space Administration (NASA), the National Science Foundation, and the U.S. Geological Survey (USGS), the National Academies of Sciences, Engineering, and Medicine established a committee to undertake the following tasks:
- Summarize current understanding of how magma is stored, ascends, and erupts.
- Discuss new disciplinary and interdisciplinary research on volcanic processes and precursors that could lead to forecasts of the type, size, and timing of volcanic eruptions.
- Describe new observations or instrument deployment strategies that could improve quantification of volcanic eruption processes and precursors.
- Identify priority research and observations needed to improve understanding of volcanic eruptions and to inform monitoring and early warning efforts.

The roles of the three agencies in advancing volcano science are summarized in Box 1.1 .
The committee held four meetings, including an international workshop, to gather information, deliberate, and prepare its report. The report is not intended to be a comprehensive review, but rather to provide a broad overview of the topics listed above. Chapter 2 addresses the opportunities for better understanding the storage, ascent, and eruption of magmas. Chapter 3 summarizes the challenges and prospects for forecasting eruptions and their consequences. Chapter 4 highlights repercussions of volcanic eruptions on a host of other Earth systems. Although not explicitly called out in the four tasks, the interactions between volcanoes and other Earth systems affect the consequences of eruptions, and offer opportunities to improve forecasting and obtain new insights into volcanic processes. Chapter 5 summarizes opportunities to strengthen
research in volcano science. Chapter 6 provides overarching conclusions. Supporting material appears in appendixes, including a list of volcano databases (see Appendix A ), a list of workshop participants (see Appendix B ), biographical sketches of the committee members (see Appendix C ), and a list of acronyms and abbreviations (see Appendix D ).
Background information on these topics is summarized in the rest of this chapter.
1.2 VOLCANOES IN THE UNITED STATES
The USGS has identified 169 potentially active volcanoes in the United States and its territories (e.g., Marianas), 55 of which pose a high threat or very high threat ( Ewert et al., 2005 ). Of the total, 84 are monitored by at least one seismometer, and only 3 have gas sensors (as of November 2016). 1 Volcanoes are found in the Cascade mountains, Aleutian arc, Hawaii, and the western interior of the continental United States ( Figure 1.4 ). The geographical extent and eruption hazards of these volcanoes are summarized below.
The Cascade volcanoes extend from Lassen Peak in northern California to Mount Meager in British Columbia. The historical record contains only small- to moderate-sized eruptions, but the geologic record reveals much larger eruptions ( Carey et al., 1995 ; Hildreth, 2007 ). Activity tends to be sporadic ( Figure 1.5 ). For example, nine Cascade eruptions occurred in the 1850s, but none occurred between 1915 and 1980, when Mount St. Helens erupted. Consequently, forecasting eruptions in the Cascades is subject to considerable uncertainty. Over the coming decades, there may be multiple eruptions from several volcanoes or no eruptions at all.
The Aleutian arc extends 2,500 km across the North Pacific and comprises more than 130 active and potentially active volcanoes. Although remote, these volcanoes pose a high risk to overflying aircraft that carry more than 30,000 passengers a day, and are monitored by a combination of ground- and space-based sensors. One or two small to moderate explosive eruptions occur in the Aleutians every year, and very large eruptions occur less frequently. For example, the world’s largest eruption of the 20th century occurred approximately 300 miles from Anchorage, in 1912.
In Hawaii, Kilauea has been erupting largely effusively since 1983, but the location and nature of eruptions can vary dramatically, presenting challenges for disaster preparation. The population at risk from large-volume, rapidly moving lava flows on the flanks of the Mauna Loa volcano has grown tremendously in the past few decades ( Dietterich and Cashman, 2014 ), and few island residents are prepared for the even larger magnitude explosive eruptions that are documented in the last 500 years ( Swanson et al., 2014 ).
All western states have potentially active volcanoes, from New Mexico, where lava flows have reached within a few kilometers of the Texas and Oklahoma borders ( Fitton et al., 1991 ), to Montana, which borders the Yellowstone caldera ( Christiansen, 1984 ). These volcanoes range from immense calderas that formed from super-eruptions ( Mastin et al., 2014 ) to small-volume basaltic volcanic fields that erupt lava flows and tephra for a few months to a few decades. Some of these eruptions are monogenic (erupt just once) and pose a special challenge for forecasting. Rates of activity in these distributed volcanic fields are low, with many eruptions during the past few thousand years (e.g., Dunbar, 1999 ; Fenton, 2012 ; Laughlin et al., 1994 ), but none during the past hundred years.
1.3 THE STRUCTURE OF A VOLCANO
Volcanoes often form prominent landforms, with imposing peaks that tower above the surrounding landscape, large depressions (calderas), or volcanic fields with numerous dispersed cinder cones, shield volcanoes, domes, and lava flows. These various landforms reflect the plate tectonic setting, the ways in which those volcanoes erupt, and the number of eruptions. Volcanic landforms change continuously through the interplay between constructive processes such as eruption and intrusion, and modification by tectonics, climate, and erosion. The stratigraphic and structural architecture of volcanoes yields critical information on eruption history and processes that operate within the volcano.
Beneath the volcano lies a magmatic system that in most cases extends through the crust, except during eruption. Depending on the setting, magmas may rise
___________________
1 Personal communication from Charles Mandeville, Program Coordinator, Volcano Hazards Program, U.S. Geological Survey, on November 26, 2016.
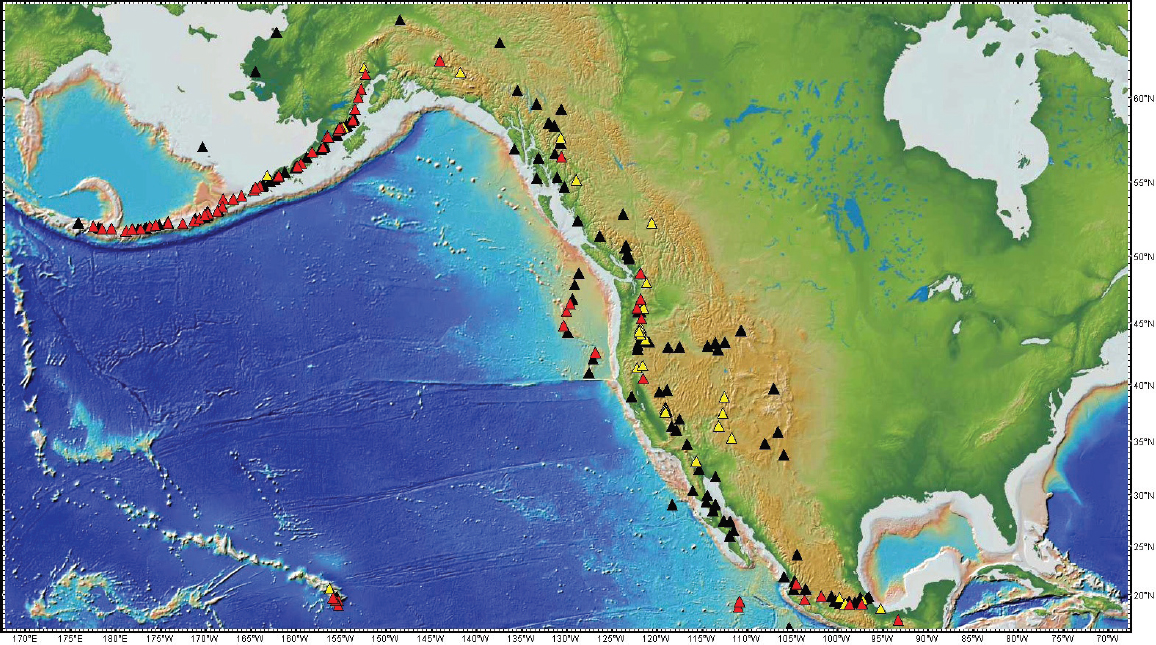
directly from the mantle or be staged in one or more storage regions within the crust before erupting. The uppermost part (within 2–3 km of Earth’s surface) often hosts an active hydrothermal system where meteoric groundwater mingles with magmatic volatiles and is heated by deeper magma. Identifying the extent and vigor of hydrothermal activity is important for three reasons: (1) much of the unrest at volcanoes occurs in hydrothermal systems, and understanding the interaction of hydrothermal and magmatic systems is important for forecasting; (2) pressure buildup can cause sudden and potentially deadly phreatic explosions from the hydrothermal system itself (such as on Ontake, Japan, in 2014), which, in turn, can influence the deeper magmatic system; and (3) hydrothermal systems are energy resources and create ore deposits.
Below the hydrothermal system lies a magma reservoir where magma accumulates and evolves prior to eruption. Although traditionally modeled as a fluid-filled cavity, there is growing evidence that magma reservoirs may comprise an interconnected complex of vertical and/or horizontal magma-filled cracks, or a partially molten mush zone, or interleaved lenses of magma and solid material ( Cashman and Giordano, 2014 ). In arc volcanoes, magma chambers are typically located 3–6 km below the surface. The magma chamber is usually connected to the surface via a fluid-filled conduit only during eruptions. In some settings, magma may ascend directly from the mantle without being stored in the crust.
In the broadest sense, long-lived magma reservoirs comprise both eruptible magma (often assumed to contain less than about 50 percent crystals) and an accumulation of crystals that grow along the margins or settle to the bottom of the magma chamber. Physical segregation of dense crystals and metals can cause the floor of the magma chamber to sag, a process balanced by upward migration of more buoyant melt. A long-lived magma chamber can thus become increasingly stratified in composition and density.
The deepest structure beneath volcanoes is less well constrained. Swarms of low-frequency earthquakes at mid- to lower-crustal depths (10–40 km) beneath volcanoes suggest that fluid is periodically transferred into the base of the crust ( Power et al., 2004 ). Tomographic studies reveal that active volcanic systems have deep crustal roots that contain, on average, a small fraction of melt, typically less than 10 percent. The spatial distribution of that melt fraction, particularly how much is concentrated in lenses or in larger magma bodies, is unknown. Erupted samples preserve petrologic and geochemical evidence of deep crystallization, which requires some degree of melt accumulation. Seismic imaging and sparse outcrops suggest that the proportion of unerupted solidified magma relative to the surrounding country rock increases with depth and that the deep roots of volcanoes are much more extensive than their surface expression.
1.4 MONITORING VOLCANOES
Volcano monitoring is critical for hazard forecasts, eruption forecasts, and risk mitigation. However, many volcanoes are not monitored at all, and others are monitored using only a few types of instruments. Some parameters, such as the mass, extent, and trajectory of a volcanic ash cloud, are more effectively measured by satellites. Other parameters, notably low-magnitude earthquakes and volcanic gas emissions that may signal an impending eruption, require ground-based monitoring on or close to the volcanic edifice. This section summarizes existing and emerging technologies for monitoring volcanoes from the ground and from space.
Monitoring Volcanoes on or Near the Ground
Ground-based monitoring provides data on the location and movement of magma. To adequately capture what is happening inside a volcano, it is necessary to obtain a long-term and continuous record, with periods spanning both volcanic quiescence and periods of unrest. High-frequency data sampling and efficient near-real-time relay of information are important, especially when processes within the volcano–magmatic–hydrothermal system are changing rapidly. Many ground-based field campaigns are time intensive and can be hazardous when volcanoes are active. In these situations, telemetry systems permit the safe and continuous collection of data, although the conditions can be harsh and the lifetime of instruments can be limited in these conditions.
Ground-based volcano monitoring falls into four broad categories: seismic, deformation, gas, and thermal monitoring ( Table 1.1 ). Seismic monitoring tools,
TABLE 1.1 Ground-Based Instrumentation for Monitoring Volcanoes
including seismometers and infrasound sensors, are used to detect vibrations caused by breakage of rock and movement of fluids and to assess the evolution of eruptive activity. Ambient seismic noise monitoring can image subsurface reservoirs and document changes in wave speed that may reflect stress. changes. Deformation monitoring tools, including tiltmeters, borehole strainmeters, the Global Navigation Satellite System (GNSS, which includes the Global Positioning System [GPS]), lidar, radar, and gravimeters, are used to detect the motion of magma and other fluids in the subsurface. Some of these tools, such as GNSS and lidar, are also used to detect erupted products, including ash clouds, pyroclastic density currents, and volcanic bombs. Gas monitoring tools, including a range of sensors ( Table 1.1 ), and direct sampling of gases and fluids are used to detect magma intrusions and changes in magma–hydrothermal interactions. Thermal monitoring tools, such as infrared cameras, are used to detect dome growth and lava breakouts. Continuous video or photographic observations are also commonly used and, despite their simplicity, most directly document volcanic activity. Less commonly used monitoring technologies, such as self-potential, electromagnetic techniques, and lightning detection are used to constrain fluid movement and to detect
ash clouds. In addition, unmanned aerial vehicles (e.g., aircraft and drones) are increasingly being used to collect data. Rapid sample collection and analysis is also becoming more common as a monitoring tool at volcano observatories. A schematic of ground-based monitoring techniques is shown in Figure 1.6 .
Monitoring Volcanoes from Space
Satellite-borne sensors and instruments provide synoptic observations during volcanic eruptions when collecting data from the ground is too hazardous or where volcanoes are too remote for regular observation. Repeat-pass data collected over years or decades provide a powerful means for detecting surface changes on active volcanoes. Improvements in instrument sensitivity, data availability, and the computational capacity required to process large volumes of data have led to a dramatic increase in “satellite volcano science.”
Although no satellite-borne sensor currently in orbit has been specifically designed for volcano monitoring, a number of sensors measure volcano-relevant
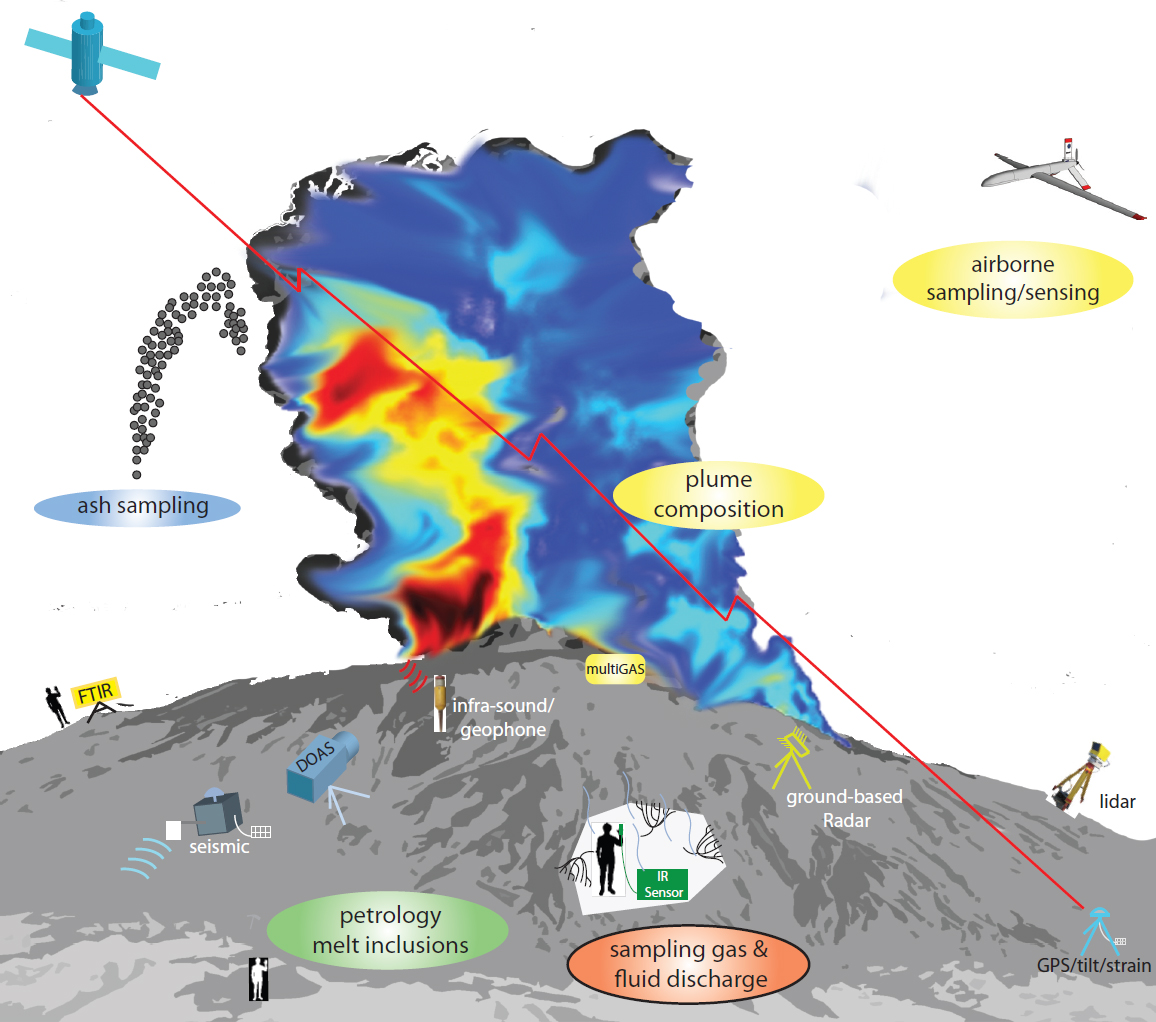
TABLE 1.2 Satellite-Borne Sensor Suite for Volcano Monitoring
NOTE: AIRS, Atmospheric Infrared Sounder; ALOS, Advanced Land Observing Satellite; ASTER, Advanced Spaceborne Thermal Emission and Reflection Radiometer; AVHRR, Advanced Very High Resolution Radiometer; CALIPSO, Cloud-Aerosol Lidar and Infrared Pathfinder Satellite Observation; COSMO-SkyMed, Constellation of Small Satellites for Mediterranean Basin Observation; GOES, Geostationary Operational Environmental Satellite; IASI, Infrared Atmospheric Sounding Interferometer; MISR, Multi-angle Imaging SpectroRadiometer; MLS, Microwave Limb Sounder; MODIS, Moderate Resolution Imaging Spectroradiometer; OMI, Ozone Monitoring Instrument; OMPS, Ozone Mapping and Profiler Suite; SAGE, Stratospheric Aerosol and Gas Experiment.
parameters, including heat flux, gas and ash emissions, and deformation ( Table 1.2 ). Thermal infrared data are used to detect eruption onset and cessation, calculate lava effusion rates, map lava flows, and estimate ash column heights during explosive eruptions. In some cases, satellites may capture thermal precursors to eruptions, although low-temperature phenomena are challenging to detect. Both high-temporal/low-spatial-resolution (geostationary orbit) and high-spatial/low-temporal-resolution (polar orbit) thermal infrared observations are needed for global volcano monitoring.
Satellite-borne sensors are particularly effective for observing the emission and dispersion of volcanic gas and ash plumes in the atmosphere. Although several volcanic gas species can be detected from space (including SO 2 , BrO, OClO, H 2 S, HCl, and CO; Carn et al., 2016 ), SO 2 is the most readily measured, and it is also responsible for much of the impact of eruptions on climate. Satellite measurements of SO 2 are valuable for detecting eruptions, estimating global volcanic fluxes and recycling of other volatile species, and tracking volcanic clouds that may be hazardous to aviation in near real time. Volcanic ash cloud altitude is most accurately determined by spaceborne lidar, although spatial coverage is limited. Techniques for measuring volcanic CO 2 from space are under development and could lead to earlier detection of preeruptive volcanic degassing.
Interferometric synthetic aperture radar (InSAR) enables global-scale background monitoring of volcano deformation ( Figure 1.7 ). InSAR provides much higher spatial resolution than GPS, but lower accuracy and temporal resolution. However, orbit repeat times will diminish as more InSAR missions are launched, such as the European Space Agency’s recently deployed Sentinel-1 satellite and the NASA–Indian Space Research Organisation synthetic aperture radar mission planned for launch in 2020.
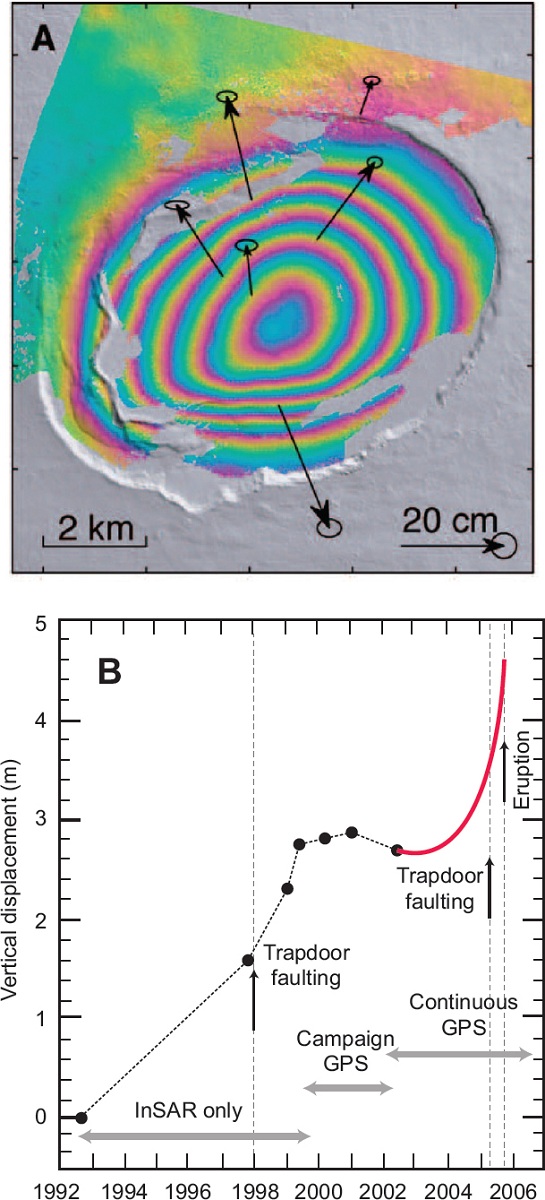
1.5 ERUPTION BEHAVIOR
Eruptions range from violently explosive to gently effusive, from short lived (hours to days) to persistent over decades or centuries, from sustained to intermittent, and from steady to unsteady ( Siebert et al., 2015 ). Eruptions may initiate from processes within the magmatic system ( Section 1.3 ) or be triggered by processes and properties external to the volcano, such as precipitation, landslides, and earthquakes. The eruption behavior of a volcano may change over time. No classification scheme captures this full diversity of behaviors (see Bonadonna et al., 2016 ), but some common schemes to describe the style, magnitude, and intensity of eruptions are summarized below.
Eruption Magnitude and Intensity
The size of eruptions is usually described in terms of total erupted mass (or volume), often referred to as magnitude, and mass eruption rate, often referred to as intensity. Pyle (2015) quantified magnitude and eruption intensity as follows:
magnitude = log 10 (mass, in kg) – 7, and
intensity = log 10 (mass eruption rate, in kg/s) + 3.
The Volcano Explosivity Index (VEI) introduced by Newhall and Self (1982) assigns eruptions to a VEI class based primarily on measures of either magnitude (erupted mass or volume) or intensity (mass eruption rate and/or eruption plume height), with more weight given to magnitude. The VEI classes are summarized in Figure 1.8 . The VEI classification is still in use, despite its many limitations, such as its reliance on only a few types of measurements and its poor fit for small to moderate eruptions (see Bonadonna et al., 2016 ).
Smaller VEI events are relatively common, whereas larger VEI events are exponentially less frequent ( Siebert et al., 2015 ). For example, on average about three VEI 3 eruptions occur each year, whereas there is a 5 percent chance of a VEI 5 eruption and a 0.2 percent chance of a VEI 7 (e.g., Crater Lake, Oregon) event in any year.
Eruption Style
The style of an eruption encompasses factors such as eruption duration and steadiness, magnitude, gas flux, fountain or column height, and involvement of magma and/or external source of water (phreatic and phreatomagmatic eruptions). Eruptions are first divided into effusive (lava producing) and explosive (pyroclast producing) styles, although individual eruptions can be simultaneously effusive and weakly explosive, and can pass rapidly and repeatedly between eruption styles. Explosive eruptions are further subdivided into styles that are sustained on time scales of hours to days and styles that are short lived ( Table 1.3 ).
Classification of eruption style is often qualitative and based on historical accounts of characteristic eruptions from type-volcanoes. However, many type-volcanoes exhibit a range of eruption styles over time (e.g., progressing between Strombolian, Vulcanian, and Plinian behavior; see Fee et al., 2010 ), which has given rise to terms such as subplinian or violent Strombolian.
1.6 ERUPTION HAZARDS
Eruption hazards are diverse ( Figure 1.9 ) and may extend more than thousands of kilometers from an active volcano. From the perspective of risk and impact, it is useful to distinguish between near-source and distal hazards. Near-source hazards are far more unpredictable than distal hazards.
Near-source hazards include those that are airborne, such as tephra fallout, volcanic gases, and volcanic projectiles, and those that are transported laterally on or near the ground surface, such as pyroclastic density currents, lava flows, and lahars. Pyroclastic density currents are hot volcanic flows containing mixtures of gas and micron- to meter-sized volcanic particles. They can travel at velocities exceeding 100 km per hour. The heat combined with the high density of material within these flows obliterates objects in their path, making them the most destructive of volcanic hazards. Lava flows also destroy everything in their path, but usually move slowly enough to allow people to get out of the way. Lahars are mixtures of volcanic debris, sediment, and water that can travel many tens of kilometers along valleys and river channels. They may be triggered during an eruption by interaction between volcanic prod-
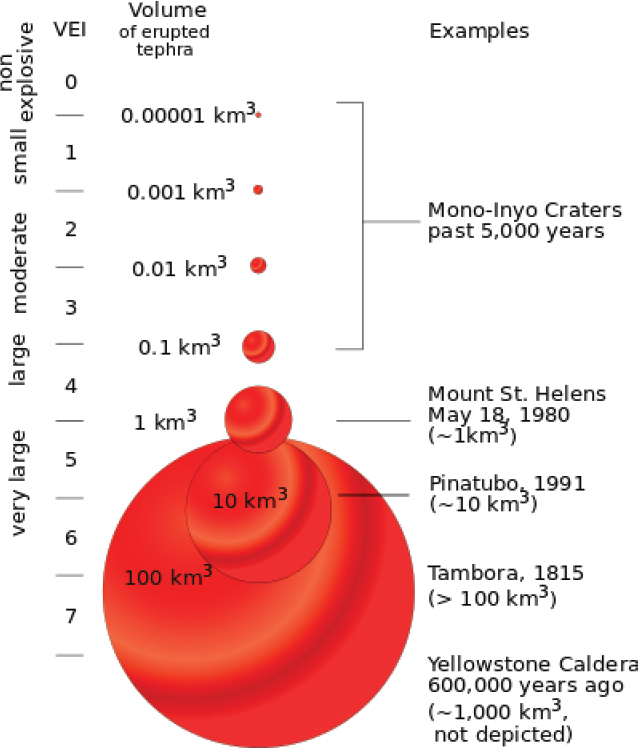
TABLE 1.3 Characteristics of Different Eruption Styles
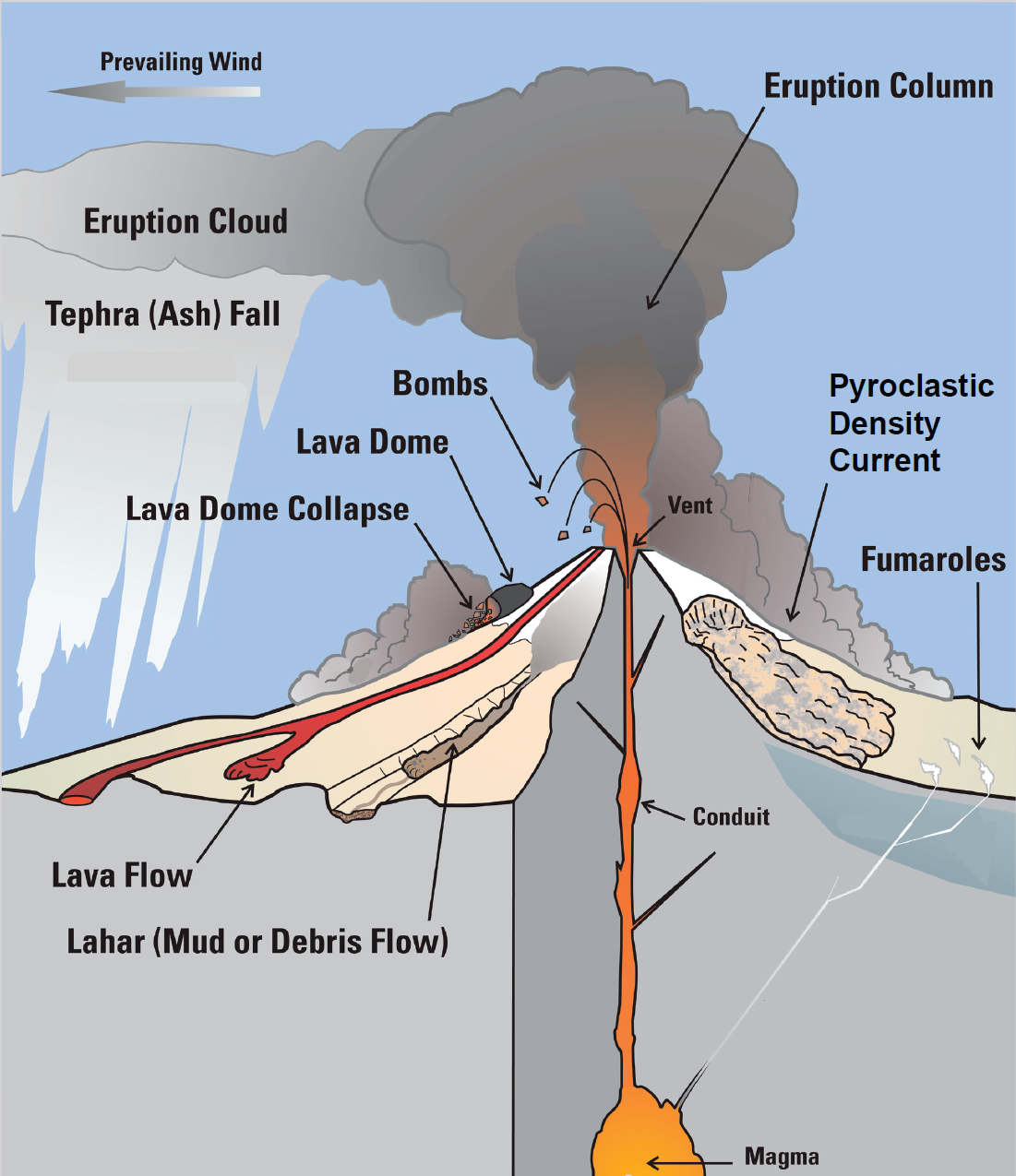
ucts and snow, ice, rain, or groundwater. Lahars can be more devastating than the eruption itself. Ballistic blocks are large projectiles that typically fall within 1–5 km from vents.
The largest eruptions create distal hazards. Explosive eruptions produce plumes that are capable of dispersing ash hundreds to thousands of kilometers from the volcano. The thickness of ash deposited depends on the intensity and duration of the eruption and the wind direction. Airborne ash and ash fall are the most severe distal hazards and are likely to affect many more people than near-source hazards. They cause respiratory problems and roof collapse, and also affect transport networks and infrastructure needed to support emergency response. Volcanic ash is a serious risk to air traffic. Several jets fully loaded with passengers have temporarily lost power on all engines after encountering dilute ash clouds (e.g., Guffanti et al., 2010 ). Large lava flows, such as the 1783 Laki eruption in Iceland, emit volcanic gases that create respiratory problems and acidic rain more than 1,000 km from the eruption. Observed impacts of basaltic eruptions in Hawaii and Iceland include regional volcanic haze (“vog”) and acid rain that affect both agriculture and human health (e.g., Thordarson and Self, 2003 ) and fluorine can contaminate grazing land and water supplies (e.g., Cronin et al., 2003 ). Diffuse degassing of CO 2 can lead to deadly concentrations with fatal consequences such as occurred at Mammoth Lakes, California, or cause lakes to erupt, leading to massive CO 2 releases that suffocate people (e.g., Lake Nyos, Cameroon).
Secondary hazards can be more devastating than the initial eruption. Examples include lahars initiated by storms, earthquakes, landslides, and tsunamis from eruptions or flank collapse; volcanic ash remobilized by wind to affect human health and aviation for extended periods of time; and flooding because rain can no longer infiltrate the ground.
1.7 MODELING VOLCANIC ERUPTIONS
Volcanic processes are governed by the laws of mass, momentum, and energy conservation. It is possible to develop models for magmatic and volcanic phenomena based on these laws, given sufficient information on mechanical and thermodynamic properties of the different components and how they interact with each other. Models are being developed for all processes in volcanic systems, including melt transport in the mantle, the evolution of magma bodies within the crust, the ascent of magmas to the surface, and the fate of magma that erupts effusively or explosively.
A central challenge for developing models is that volcanic eruptions are complex multiphase and multicomponent systems that involve interacting processes over a wide range of length and time scales. For example, during storage and ascent, the composition, temperature, and physical properties of magma and host rocks evolve. Bubbles and crystals nucleate and grow in this magma and, in turn, greatly influence the properties of the magmas and lavas. In explosive eruptions, magma fragmentation creates a hot mixture of gas and particles with a wide range of sizes and densities. Magma also interacts with its surroundings: the deformable rocks that surround the magma chamber and conduit, the potentially volatile groundwater and surface water, a changing landscape over which pyroclastic density currents and lava flows travel, and the atmosphere through which eruption columns rise.
Models for volcanic phenomena that involve a small number of processes and that are relatively amenable to direct observation, such as volcanic plumes, are relatively straightforward to develop and test. In contrast, phenomena that occur underground are more difficult to model because there are more interacting processes. In those cases, direct validation is much more challenging and in many cases impossible. Forecasting ash dispersal using plume models is more straightforward and testable than forecasting the onset, duration, and style of eruption using models that seek to explain geophysical and geochemical precursors. In all cases, however, the use of even imperfect models helps improve the understanding of volcanic systems.
Modeling approaches can be divided into three categories:
- Reduced models make simplifying assumptions about dynamics, heat transfer, and geometry to develop first-order explanations for key properties and processes, such as the velocity of lava flows and pyroclastic density currents, the height of eruption columns, the magma chamber size and depth, the dispersal of tephra, and the ascent of magma in conduits. Well-calibrated or tested reduced models offer a straightforward ap-
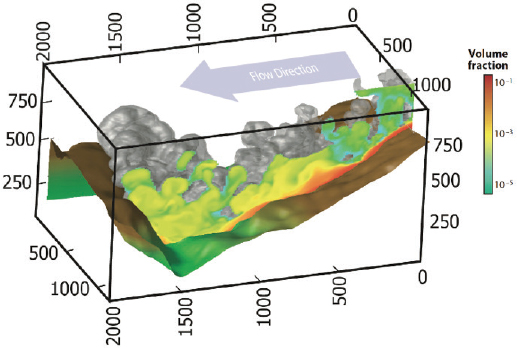
proach for combining observations and models in real time in an operational setting (e.g., ash dispersal forecasting for aviation safety). Models may not need to be complex if they capture the most important processes, although simplifications require testing against more comprehensive models and observations.
- Multiphase and multiphysics models improve scientific understanding of complex processes by invoking fewer assumptions and idealizations than reduced models ( Figure 1.10 ), but at the expense of increased complexity and computational demands. They also require additional components, such as a model for how magma in magma chambers and conduits deforms when stressed; a model for turbulence in pyroclastic density currents and plumes; terms that describe the thermal and mechanical exchange among gases, crystals, and particles; and a description of ash aggregation in eruption columns. A central challenge for multiphysics models is integrating small-scale processes with large-scale dynamics. Many of the models used in volcano science build on understanding developed in other science and engineering fields and for other ap-
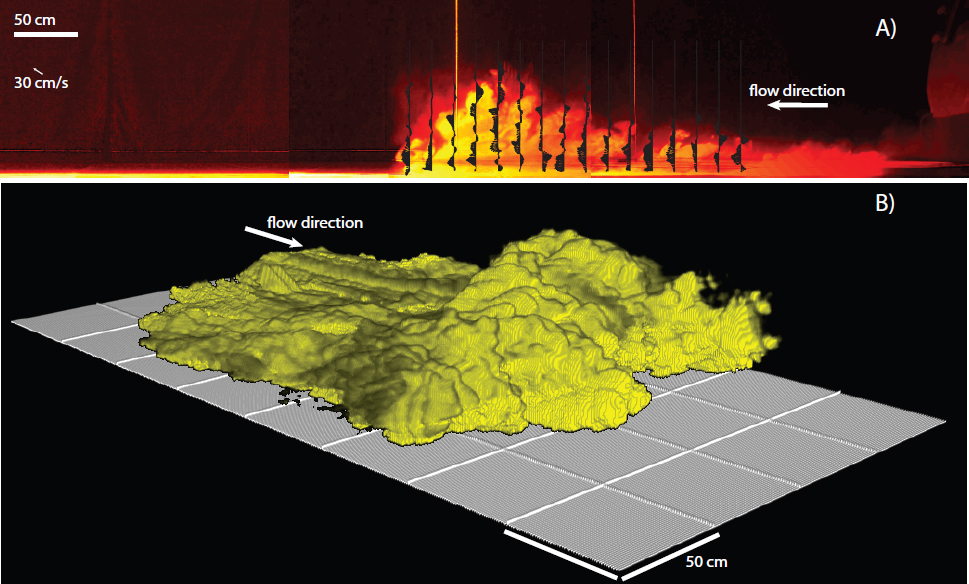
plications. Multiphysics and multiscale models benefit from rapidly expanding computational capabilities.
- Laboratory experiments simulate processes for which the geometry and physical and thermal processes and properties can be scaled ( Mader et al., 2004 ). Such experiments provide insights on fundamental processes, such as crystal dynamics in flowing magmas, entrainment in eruption columns, propagation of dikes, and sedimentation from pyroclastic density currents ( Figure 1.11 ). Experiments have also been used successfully to develop the subsystem models used in numerical simulations, and to validate computer simulations for known inputs and properties.
The great diversity of existing models reflects to a large extent the many interacting processes that operate in volcanic eruptions and the corresponding simplifying assumptions currently required to construct such models. The challenge in developing models is often highlighted in discrepancies between models and observations of natural systems. Nevertheless, eruption models reveal essential processes governing volcanic eruptions, and they provide a basis for interpreting measurements from prehistoric and active eruptions and for closing observational gaps. Mathematical models offer a guide for what observations will be most useful. They may also be used to make quantitative and testable predictions, supporting forecasting and hazard assessment.
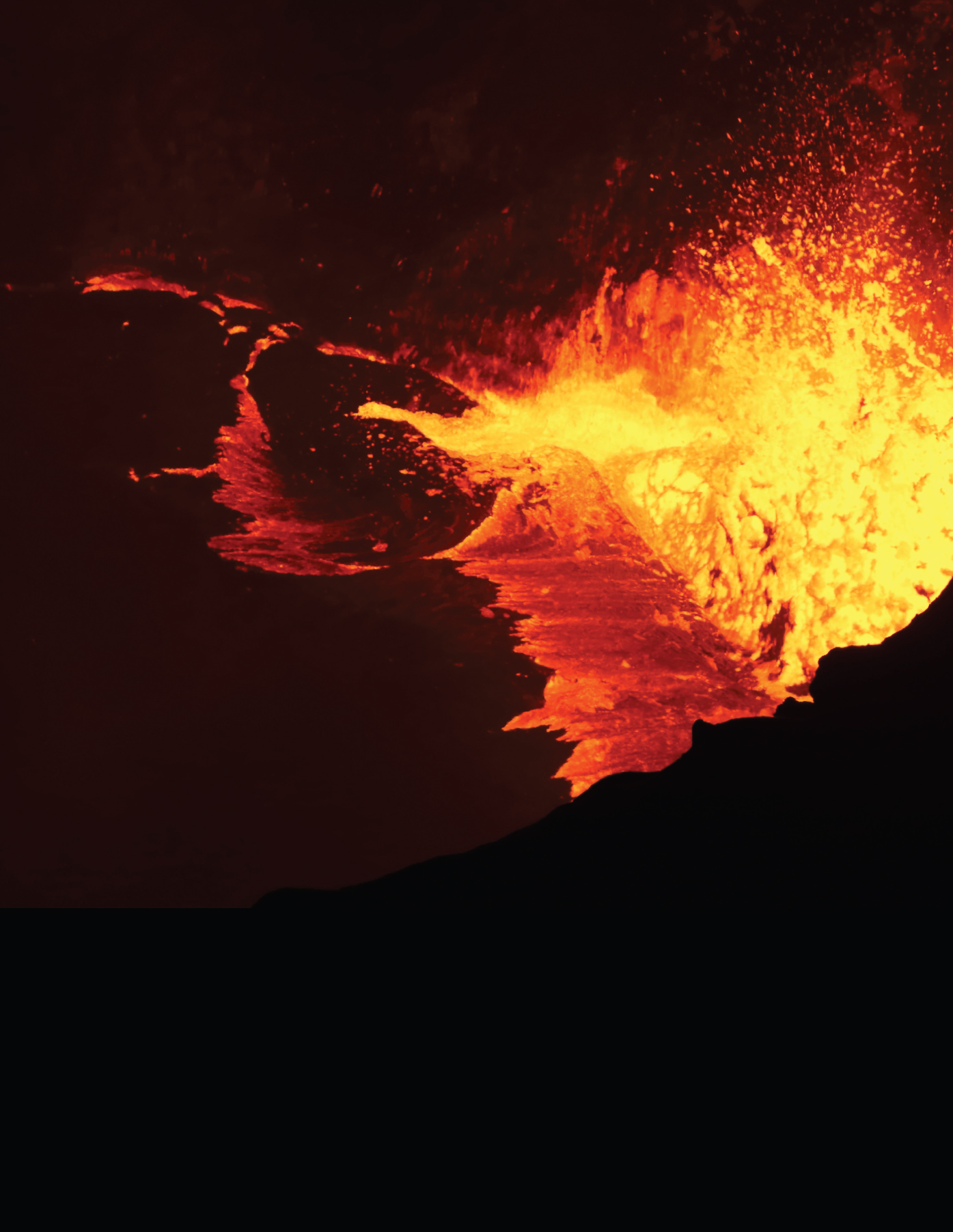
Volcanic eruptions are common, with more than 50 volcanic eruptions in the United States alone in the past 31 years. These eruptions can have devastating economic and social consequences, even at great distances from the volcano. Fortunately many eruptions are preceded by unrest that can be detected using ground, airborne, and spaceborne instruments. Data from these instruments, combined with basic understanding of how volcanoes work, form the basis for forecasting eruptions—where, when, how big, how long, and the consequences.
Accurate forecasts of the likelihood and magnitude of an eruption in a specified timeframe are rooted in a scientific understanding of the processes that govern the storage, ascent, and eruption of magma. Yet our understanding of volcanic systems is incomplete and biased by the limited number of volcanoes and eruption styles observed with advanced instrumentation. Volcanic Eruptions and Their Repose, Unrest, Precursors, and Timing identifies key science questions, research and observation priorities, and approaches for building a volcano science community capable of tackling them. This report presents goals for making major advances in volcano science.
READ FREE ONLINE
Welcome to OpenBook!
You're looking at OpenBook, NAP.edu's online reading room since 1999. Based on feedback from you, our users, we've made some improvements that make it easier than ever to read thousands of publications on our website.
Do you want to take a quick tour of the OpenBook's features?
Show this book's table of contents , where you can jump to any chapter by name.
...or use these buttons to go back to the previous chapter or skip to the next one.
Jump up to the previous page or down to the next one. Also, you can type in a page number and press Enter to go directly to that page in the book.
Switch between the Original Pages , where you can read the report as it appeared in print, and Text Pages for the web version, where you can highlight and search the text.
To search the entire text of this book, type in your search term here and press Enter .
Share a link to this book page on your preferred social network or via email.
View our suggested citation for this chapter.
Ready to take your reading offline? Click here to buy this book in print or download it as a free PDF, if available.
Get Email Updates
Do you enjoy reading reports from the Academies online for free ? Sign up for email notifications and we'll let you know about new publications in your areas of interest when they're released.
How Volcanoes Influence Climate
Volcanic eruptions are responsible for releasing molten rock, or lava, from deep within the Earth, forming new rock on the Earth’s surface. But the largest and most explosive eruptions also impact the atmosphere.
The gases and dust particles thrown into the atmosphere during large volcanic eruptions can influence climate. Particles spewed from volcanoes, like dust and ash, can cause temporary cooling by shading incoming solar radiation if the particles were launched high enough into the atmosphere. The cooling effect can last for months to years depending on the eruption. But volcanoes can also cause climate to warm when eruptions spew greenhouse gases into the atmosphere. Over millions of years this caused global warming during times in Earth’s history when extreme amounts of volcanism emitted large amounts of greenhouse gases.
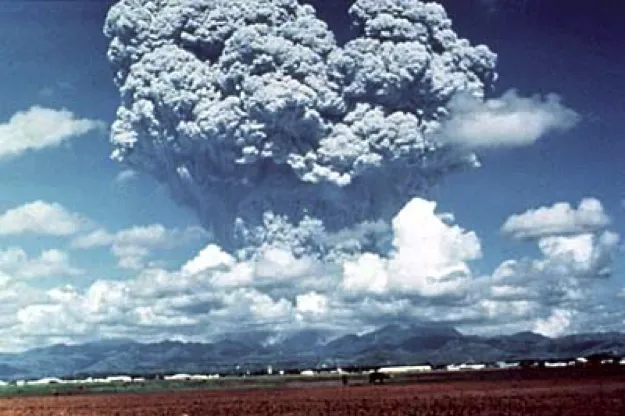
A huge cloud of volcanic ash and gas rises above Mount Pinatubo, Philippines, on June 12, 1991. Three days later, the volcano exploded in the second-largest volcanic eruption on Earth in the 20th century.
Even though volcanoes are in specific places on Earth, their effects can be more widely distributed as gases, dust, and ash get into the atmosphere. Because of atmospheric circulation patterns, eruptions in the tropics can have an effect on the climate in both hemispheres while eruptions at mid or high latitudes only have impact the hemisphere they are within.
Below is an overview of materials that make their way from volcanic eruptions into the atmosphere: particles of dust and ash, sulfur dioxide, and greenhouse gases like water vapor and carbon dioxide.
Particles of Dust and Ash
Volcanic ash or dust released into the atmosphere during an eruption shade sunlight and cause temporary cooling. Larger particles of ash have little effect because they fall out of the air quickly. Small ash particles form a dark cloud in the troposphere that shades and cools the area directly below. Most of these particles fall out of the atmosphere within rain a few hours or days after an eruption. But the smallest particles of dust get into the stratosphere and are able to travel vast distances, often worldwide. These tiny particles are so light that they can stay in the stratosphere for months, blocking sunlight and causing cooling over large areas of the Earth.
Sulfur from Volcanoes
Often, erupting volcanoes emit sulfur dioxide into the atmosphere. Sulfur dioxide is much more effective than ash particles at cooling the climate. The sulfur dioxide moves into the stratosphere and combines with water to form sulfuric acid aerosols. The sulfuric acid makes a haze of tiny droplets in the stratosphere that reflects incoming solar radiation, causing cooling of the Earth’s surface. The aerosols can stay in the stratosphere for up to three years, moved around by winds and causing significant cooling worldwide. Eventually, the droplets grow large enough to fall to Earth.
Greenhouse Gases Emitted by Volcanoes
Volcanoes also release large amounts of greenhouse gases such as water vapor and carbon dioxide. The amounts put into the atmosphere from a large eruption doesn't change the global amounts of these gases very much. However, there have been times during Earth history when intense volcanism has significantly increased the amount of carbon dioxide in the atmosphere and caused global warming.
- How the Geosphere Rocks Climate
- Volcanic Gases and Climate Change (USGS)
- How do volcanoes affect world climate? (Scientific American)
- What is Climate?
Related Links
- Measuring the Climate Effects of Volcanic Eruptions
November 29, 1999
What Causes a Volcano to Erupt, and How Do Scientists Predict Eruptions?
Volcanologists cannot yet predict a volcanic eruption
By Attila Kilinc
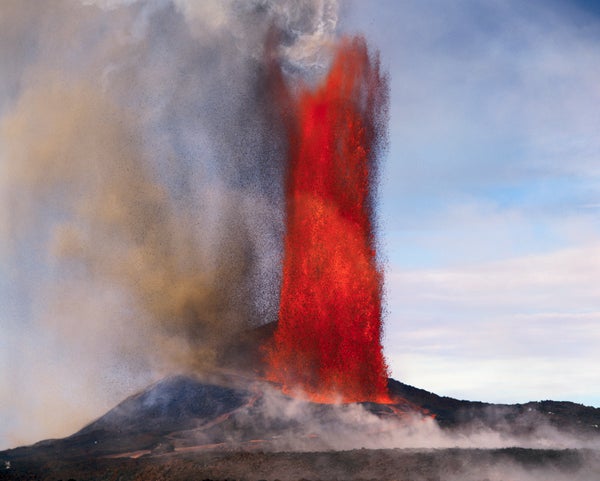
Kilauea erupting.
Douglas Peebles Getty Images
Editor’s Note (6/4/18): This story is being re-posted in light of the deadly eruption of Guatemala’s Fuego volcano on Sunday (June 3), which covered nearby villages in fast-moving ash flows.
Attila Kilinc, head of the geology department at the University of Cincinnati, offers this answer. Most recently, Professor Kilinc has been studying volcanoes in Hawaii and Montserrat.
When a part of the earth's upper mantle or lower crust melts, magma forms. A volcano is essentially an opening or a vent through which this magma and the dissolved gases it contains are discharged. Although there are several factors triggering a volcanic eruption, three predominate: the buoyancy of the magma, the pressure from the exsolved gases in the magma and the injection of a new batch of magma into an already filled magma chamber. What follows is a brief description of these processes.
On supporting science journalism
If you're enjoying this article, consider supporting our award-winning journalism by subscribing . By purchasing a subscription you are helping to ensure the future of impactful stories about the discoveries and ideas shaping our world today.
As rock inside the earth melts, its mass remains the same while its volume increases--producing a melt that is less dense than the surrounding rock. This lighter magma then rises toward the surface by virtue of its buoyancy. If the density of the magma between the zone of its generation and the surface is less than that of the surrounding and overlying rocks, the magma reaches the surface and erupts.
Magmas of so-called andesitic and rhyolitic compositions also contain dissolved volatiles such as water, sulfur dioxide and carbon dioxide. Experiments have shown that the amount of a dissolved gas in magma (its solubility) at atmospheric pressure is zero, but rises with increasing pressure.
For example, in an andesitic magma saturated with water and six kilometers below the surface, about 5 percent of its weight is dissolved water. As this magma moves toward the surface, the solubility of the water in the magma decreases, and so the excess water separates from the magma in the form of bubbles. As the magma moves closer to the surface, more and more water exsolves from the magma, thereby increasing the gas/magma ratio in the conduit. When the volume of bubbles reaches about 75 percent, the magma disintegrates to pyroclasts (partially molten and solid fragments) and erupts explosively.
The third process that causes volcanic eruptions is an injection of new magma into a chamber that is already filled with magma of similar or different composition. This injection forces some of the magma in the chamber to move up in the conduit and erupt at the surface.
Although volcanologists are well aware of these three processes, they cannot yet predict a volcanic eruption. But they have made significant advances in forecasting volcanic eruptions. Forecasting involves probable character and time of an eruption in a monitored volcano. The character of an eruption is based on the prehistoric and historic record of the volcano in question and its volcanic products. For example, a violently erupting volcano that has produced ash fall, ash flow and volcanic mudflows (or lahars) is likely to do the same in the future.
Determining the timing of an eruption in a monitored volcano depends on measuring a number of parameters, including, but not limited to, seismic activity at the volcano (especially depth and frequency of volcanic earthquakes), ground deformations (determined using a tiltmeter and/or GPS, and satellite interferometry), and gas emissions (sampling the amount of sulfur dioxide gas emitted by correlation spectrometer, or COSPEC). An excellent example of successful forecasting occurred in 1991. Volcanologists from the U.S. Geological Survey accurately predicted the June 15 eruption of the Pinatubo Volcano in the Philippines, allowing for the timely evacuation of the Clark Air Base and saving thousands of lives.
Advertisement
Volcanic air pollution and human health: recent advances and future directions
- Perspectives
- Open access
- Published: 21 December 2021
- Volume 84 , article number 11 , ( 2022 )
Cite this article
You have full access to this open access article
- Carol Stewart ORCID: orcid.org/0000-0002-3781-6308 1 ,
- David E. Damby ORCID: orcid.org/0000-0002-3238-3961 2 ,
- Claire J. Horwell ORCID: orcid.org/0000-0002-0148-6933 3 ,
- Tamar Elias ORCID: orcid.org/0000-0002-9592-4518 4 ,
- Evgenia Ilyinskaya ORCID: orcid.org/0000-0002-3663-9506 5 ,
- Ines Tomašek ORCID: orcid.org/0000-0003-3743-6628 6 , 7 ,
- Bernadette M. Longo ORCID: orcid.org/0000-0003-0502-3749 8 ,
- Anja Schmidt ORCID: orcid.org/0000-0001-8759-2843 9 , 10 ,
- Hanne Krage Carlsen ORCID: orcid.org/0000-0003-1656-9624 11 , 12 ,
- Emily Mason ORCID: orcid.org/0000-0002-7050-6475 13 ,
- Peter J. Baxter ORCID: orcid.org/0000-0001-8700-1249 14 ,
- Shane Cronin ORCID: orcid.org/0000-0001-7499-603X 15 &
- Claire Witham ORCID: orcid.org/0000-0001-5037-6987 16
14k Accesses
34 Citations
14 Altmetric
Explore all metrics
Volcanic air pollution from both explosive and effusive activity can affect large populations as far as thousands of kilometers away from the source, for days to decades or even centuries. Here, we summarize key advances and prospects in the assessment of health hazards, effects, risk, and management. Recent advances include standardized ash assessment methods to characterize the multiple physicochemical characteristics that might influence toxicity; the rise of community-based air quality monitoring networks using low-cost gas and particulate sensors; the development of forecasting methods for ground-level concentrations and associated public advisories; the development of risk and impact assessment methods to explore health consequences of future eruptions; and the development of evidence-based, locally specific measures for health protection. However, it remains problematic that the health effects of many major and sometimes long-duration eruptions near large populations have gone completely unmonitored. Similarly, effects of prolonged degassing on exposed populations have received very little attention relative to explosive eruptions. Furthermore, very few studies have longitudinally followed populations chronically exposed to volcanic emissions; thus, knowledge gaps remain about whether chronic exposures can trigger development of potentially fatal diseases. Instigating such studies will be facilitated by continued co-development of standardized protocols, supporting local study teams and procuring equipment, funding, and ethical permissions. Relationship building between visiting researchers and host country academic, observatory, and agency partners is vital and can, in turn, support the effective communication of health impacts of volcanic air pollution to populations, health practitioners, and emergency managers.
Similar content being viewed by others
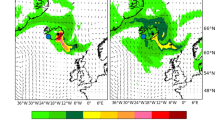
Increased respiratory morbidity associated with exposure to a mature volcanic plume from a large Icelandic fissure eruption
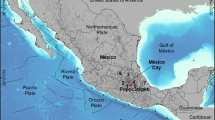
Ash emission from a long-lived eruption at Popocatépetl volcano and mapped respiratory effects
Air quality in lanzhou, a major industrial city in china: characteristics of air pollution and review of existing evidence from air pollution and health studies.
Avoid common mistakes on your manuscript.
Introduction
Globally, over a billion people are estimated to live within 100 km of an active volcano (Freire et al. 2019 ). Volcanic eruptions may cause injuries and fatalities via a range of hazardous phenomena (e.g., pyroclastic density currents, ballistics, lahars, lava flows, and localized accumulations or flows of asphyxiant gases such as CO 2 and H 2 S), affecting communities within tens of kilometers of the vent (Brown et al. 2017 ). Eruptions may also displace large numbers of people temporarily or permanently (Cuthbertson et al. 2020 ) with cascading health and social impacts including disease outbreaks due to overcrowding, food insecurity, mental health issues, and violence (Connell and Lutkehaus 2017 ). Airborne volcanic emissions, often referred to as “volcanic air pollution” (Tam et al. 2016 ; Crawford et al. 2021 ), can also present chronic, far-reaching hazards which may have harmful and long-lasting effects on populations across large geographic areas (Oppenheimer et al. 2003 ). Here, we address the state of knowledge regarding volcanic air pollution and health. This includes a discussion of hazard assessment methods, a summary of reported human health effects, a review of risk assessment, population preparedness and protection practices, and a discussion of emerging themes and future directions.
Volcanic emission hazards
Airborne volcanic emissions comprise variable mixtures of silicate ash, gases (H 2 O, CO 2 , SO 2 , H 2 S, CO, HF, and HCl), volatile metal vapors, and sulfate aerosol, formed through SO 2 gas-to-particle conversion (Fig. 1 ; Oppenheimer et al. 2003 ). Ash can be generated during a variety of eruptive processes and can contain substantial amounts of respirable-sized particles (<4 μm diameter) that can penetrate into the lungs (Horwell 2007 ). The physical and chemical properties of ash can vary significantly across eruptions and with distance (Jenkins et al. 2015 ). As volcanic gases cool and react in the atmosphere, they may condense into particles and/or adsorb to ash surfaces (Oppenheimer et al. 2003 ). Volcanic aerosol particles formed through gas condensation are extremely fine-grained, typically ~0.2–0.5 μm in diameter (Mather et al. 2003 ). Volcanic particulate matter (PM) thus encompasses a heterogeneous mixture of ash PM and acidic sulfate- and metal-bearing aerosol PM. A further airborne hazard is generated when lava flows into seawater, generating a “laze” (lava + haze) plume that contains HCl, volcanic glass fragments, and various metals (Mason et al. 2021 ; Fig. 1 ).
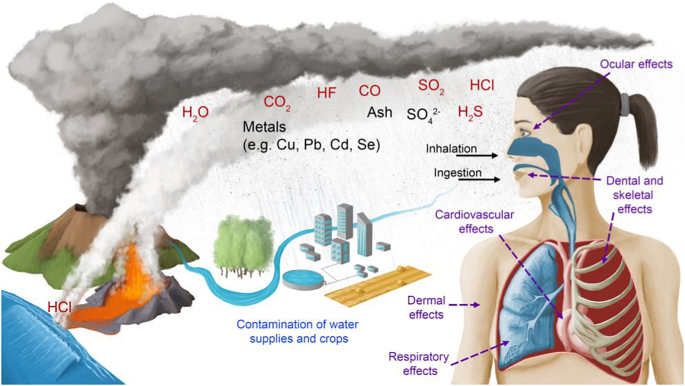
Volcanic emission components, exposure pathways, and categories of human health effects. Gaseous species are shown in red and particulate species in black. The upper plume represents airborne emissions from an explosive eruption, while the lower plume is generated by effusive activity. A steamy “laze” plume, created by interaction of molten lava with seawater, contains hydrochloric acid, various metals, and volcanic glass particles. Important plume processes include gas to particle conversion and adsorption of gas onto silicate ash surfaces. Contamination of water supplies by volcanogenic fluoride (from gaseous HF) is proposed to be the major ingestion pathway leading to human dental and skeletal effects.
Sulfur gases (in particular SO 2 ), sulfate aerosol, and ash are the most important airborne hazards for population-scale, longer-term impacts and have been shown to affect air quality locally as well as hundreds to thousands of kilometers from source during large fissure or explosive eruptions (e.g., Schmidt et al. 2011 , 2015 ; de Lima et al. 2012 ; Durant et al. 2012 ; Eychenne et al. 2015 ; Ilyinskaya et al. 2017 ). Many of the volatile trace elements emitted by volcanoes are classified as metal pollutants by environmental and health protection agencies (e.g., lead, zinc, arsenic, cadmium), Footnote 1 and emission rates can reach levels comparable to anthropogenic fluxes from industrialized countries (Ilyinskaya et al. 2021 ). Near persistently degassing volcanoes, elevated levels of metals have been reported in air, soils, surface waters, and plants (Delmelle 2003 ), which are common exposure sources for humans (Prüss-Ustün et al. 2011 ), especially in areas where communities consume catchment or surface water and locally grown crops. Persistent degassing is also the source of fluoride contamination of water resources close to certain volcanoes, notably Ambrym and Tanna, Vanuatu (Cronin and Sharp 2002 ; Allibone et al. 2012 ; Webb et al. 2021 ). Acidified rainfall from persistent degassing can leach lead from plumbing fittings or roofing materials into roof catchment rainwater tanks (Macomber 2020 ). Ash deposition into water supplies can raise concentrations of fluoride and other potentially toxic elements (e.g., copper, manganese) as well as elements that impart an unpleasant taste or color to the water (Stewart et al. 2006 , 2020 ).
Hazard and exposure assessment
In an eruption crisis, it is rare for there to be an immediate assessment of the health impact of exposure to volcanic air pollution. With limited resources, health agencies must prioritize ensuring sanitary conditions for evacuated communities and monitoring these communities for infectious disease outbreaks, as well as dealing with casualties. In lieu of data to directly measure the health impact, the physicochemical characteristics of the emissions, along with exposure concentrations and durations, may be assessed to get a first indication of whether they may be hazardous to human health.
For volcanic ash, characteristics that inform whether ash may cause harm if inhaled or ingested include particle size, particle shape, surface area, and the presence of leachable elements. Additional, specific hazards can vary according to magma composition and eruption dynamics. For lava dome-related or intermediate to felsic explosive ash samples, crystalline silica (quartz and its polymorphs) is important to quantify as it is the mineral of greatest health concern in ash due to its capacity to cause disease in industrial settings (Baxter et al. 1999 ; Greenberg et al. 2007 ). For mafic samples, reactive surface iron and associated generation of free radicals, which are implicated in respiratory diseases (Kelly 2003 ), can be determined (Horwell et al. 2007 ). Leachate analyses can determine concentrations of readily soluble elements on fresh ash particles relevant to inhalation or ingestion pathways. These methods may require adaptation for ash from hydrothermal system eruptions which typically contain fluoride in slowly soluble forms (Cronin et al. 2014 ; Stewart et al. 2020 ). Ash can also scavenge biologically potent organic pollutants from the atmosphere (Tomašek et al. 2021a ). Toxicological assays can be used to assess whether the ash can trigger a biological response, which gives an indication of potential pathogenicity for humans (Damby et al. 2016 ).
The International Volcanic Health Hazard Network (IVHHN) Footnote 2 has developed methods and protocols for rapid, standardized screening of ash samples (Le Blond et al. 2009 ; Horwell 2007 ; Horwell et al. 2007 ; Stewart et al. 2020 ; Tomašek et al. 2021b ), which have been applied during various eruption crises. Table 1 presents post-2000 studies that have determined health-relevant characteristics of ash samples and whether they have used IVHHN methods or not. The major challenges associated with ash characterization relate to timely collection of ash samples, prior capacity building and training in suitable laboratories, funding analyses, and shipping of samples, given that transportation is often disrupted during an eruption. In practice, analyses are rarely completed within the days to weeks over which acute exposures may be occurring, so cannot be relied upon to inform decision-making. Thus, in advance of future eruptions, the hazard could be informed by study of archived ash samples from historic eruptions (Hillman et al. 2012 ; Horwell et al. 2010b , 2017 ; Damby et al. 2017 ).
Exposure to volcanic emissions rarely occurs in clean atmospheres, raising concerns about co-exposures of volcanic emissions and existing air pollution, particularly in urban areas. Preliminary work on these combined hazards indicates that the specific mixture may be important, with a heightened pro-inflammatory response (in laboratory in vitro tests) reported for simultaneous exposure to respirable ash and diesel exhaust particles (Tomašek et al. 2016 ) but not for ash and complete gasoline exhaust (Tomašek et al. 2018 ).
Real-time monitoring of airborne gas and PM concentrations can be used as a proxy for assessing population exposure during eruptions, for persistent degassing, and for post-eruption ash resuspension episodes (Wilson et al. 2011 ). Indoor and outdoor measurements may be made via fixed monitors or portable sensors. Ambient air quality limits exist for airborne contaminants common to volcanic emissions such as PM 10 , PM 2.5 , and SO 2 , and monitoring data can be used to help alert both healthy and sensitive populations. However, air quality monitoring equipment is not installed at many volcanic locations, and installing instrumentation following eruption onset can present significant challenges (Felton et al. 2019 ). This can hinder agencies in making evidence-based decisions on community protection. An additional challenge to characterizing volcanic air pollution is that SO 2 and PM concentrations can vary significantly over short distances and durations (Holland et al. 2020 ). This issue has received significant attention recently with the introduction of low-cost fixed networks and hand-held, portable sensors that augment higher accuracy but costly regulatory air quality monitoring. These low-cost PM and SO 2 sensors perform reasonably well for monitoring volcanic air pollution in communities, as demonstrated during the Kīlauea 2018 eruption (Whitty et al. 2020 ; Crawford et al. 2021 ) and in Iceland (Gíslason et al. 2015 ). Air quality forecast models can complement ambient air monitoring and now play an important role in informing the public about current and predicted levels of volcanic pollution in some locations (Barsotti 2020 ; Holland et al. 2020 ).
Assessment of health effects
Post-2000 clinical and epidemiological studies conducted on communities affected by volcanic emissions are presented in Table 2 . Collectively, these studies support pre-2000 findings, from studies conducted predominantly at Mount St. Helens, Soufrière Hills, and Sakurajima, that exposures to airborne volcanic emissions can exacerbate symptoms of pre-existing lung conditions (reviewed in Horwell and Baxter 2006 ). However, very few of these studies have followed populations longitudinally using the timeframes needed (on the order of decades) for long-latency diseases such as pneumoconioses or cancers to manifest. Further, situations that produce chronic exposure to ash are rare, with the best-documented examples being the 15-year cumulative exposure to ash from Soufrière Hills volcano, Montserrat (Baxter et al. 2014 ) and the eruption of Sakurajima volcano, Japan, with frequent ash exposures since the 1970s (reviewed by Hillman et al. 2012 ). Overall, major knowledge gaps remain about whether chronic exposures can trigger development of potentially fatal cardiorespiratory diseases and also whether chronic health effects can result from acute exposures.
Beyond respiratory health, studies of human exposure to volcanic emissions have also reported on ocular, dermal, and cardiovascular effects, gastroenteritis, birth outcomes, dental fluorosis, acute injury, and increased use of healthcare services and medications (summarized in Table 2 ). Documented instances of human fluorosis associated with active volcanism are rare globally (D’Alessandro 2006 ; Table 2 ) but may be under-reported. Mental health has also received attention, but few studies have addressed specific mental health impacts resulting from exposure to volcanic air pollution.
The evidence base is weak on which characteristics of volcanic or other air pollution sources are responsible for the observed negative health outcomes. Routine monitoring does not cover all species of concern (e.g., metal pollutants and aerosol acidity). In the past decade, many air pollution studies in cities with traffic-related emissions have shown the importance of fine particulate matter, particularly PM 2.5 , in the development of certain acute and chronic health conditions (respiratory, including lung cancer, and cardiovascular, in particular) and daily mortality. A future challenge will be to determine whether this applies to volcanic PM, as the World Health Organization has concluded that these outcomes relate to geogenic as well as anthropogenic particulate exposures (World Health Organization 2013 ).
Currently we have little clinical evidence of whether chronic exposures to volcanic crystalline silica can trigger silicosis or lung cancer (World Health Organization 1997 ). Some toxicology studies have indicated biological mechanisms for silica-related diseases (Lee and Richards 2004 ; Damby et al. 2018b ). However, there is also evidence that volcanic crystalline silica may not be particularly toxic (Damby et al. 2016 ). Geochemical and crystallographic studies indicate that, as with other forms of silica dusts (International Agency for Research on Cancer 1997 ; Donaldson and Borm 1998 ), there are inherent characteristics of the silica, and external factors, which may dampen its toxicity, such as chemical (e.g. aluminum) impurities in the crystal structure or the presence of the crystalline silica within an occluding complex mineral matrix (Horwell et al. 2012 ; Damby et al. 2014 ; Nattrass et al. 2017 ).
Conducting high-quality studies on health effects is challenging during an eruption crisis, and the need is often secondary to emergency response. Consequently, important opportunities to study population exposures and health impacts have been missed. Furthermore, many countries with frequent volcanism do not routinely gather public health statistics, or they may have low-quality population registers and no exposure monitoring in place. These conditions make health assessment and follow-up even more challenging. It is also extremely difficult to follow a cohort of people over decades, especially if exposures of study participants are curtailed due to evacuation or permanent migration following the eruption. Obtaining funding for longitudinal studies and having the long-term support of local healthcare professionals and facilities are also great challenges.
Risk assessment and management
Increased knowledge about the hazards posed by volcanic emissions now enables risk assessments (also known as Health Impact Assessments; HIA) to be conducted prior to, or during, eruptions. To date, three such assessments have been published: Hincks et al. ( 2006 ), on crystalline silica-rich ash exposures on Montserrat; and Schmidt et al. ( 2011 ) and Heaviside et al. ( 2021 ) on SO 2 /sulfate exposures from a future Laki-style eruption.
Mueller et al. ( 2020a ) recently reviewed the potential for conducting HIAs in volcanic locations to predict future morbidity and mortality due to ash exposures from eruptions, given knowledge of eruption scenarios, baseline health data, and expected exposures. They concluded that, given the scarcity of published clinical/epidemiological studies and exposure data from eruptions, the application of outdoor urban air pollution risk estimates (concentration-response functions) to eruption scenarios was the best way to estimate the impact from volcanic ash exposures. Local climate, socioeconomic status, and quality of healthcare facilities also influence vulnerability and should be included in risk calculations.
Progress is being made in integrating atmospheric, volcanological, and medical information for real-time risk management. For example, detailed modeling of volcanic plume chemistry and transport from the 2014 to 2015 Holuhraun eruption informed exposure assessment (Carlsen et al. 2021a ). At Kīlauea, characterizing vog (SO 2 and aerosol concentrations) has led to improved exposure assessments for studies seeking to understand vog health impacts (Tam et al. 2016 ).
Civil protection exercises for volcanic eruptions are now starting to include volcanic emissions (Holland et al. 2020 ; Witham et al. 2020 ). Such preparedness steps will help to identify where risks from volcanism need to be balanced against other local background issues and environmental hazards.
Due to the knowledge gaps, especially those related to the health effects of chronic exposures (e.g., to crystalline silica), a precautionary approach is generally taken to the management of health risks. Many agencies around the world will advise communities to reduce their exposures to volcanic air pollution. Little data exists on the efficacy of intervention strategies (air purifiers, dehumidifiers, or air conditioners) on indoor air quality in a volcanic environment. However, recent studies have provided an evidence base for the efficacy of wearing personal respiratory protection to reduce exposure to volcanic ash (Mueller et al. 2018 ; Steinle et al. 2018 ). The finding that industry-certified N95-style masks are most effective but hard to source and afford has led to some humanitarian organizations donating or crowdfunding such masks (Horwell et al. 2020 ). However, many government agencies distribute less-effective stockpiled masks, raising important ethical questions about the morality and legality of providing suboptimal protection (McDonald et al. 2020 ; McDonald and Horwell 2021 ). Provision of information on intervention effectiveness that is specific to local climates and cultures can help address such concerns. For example, IVHHN has produced informational products on protection from volcanic emissions, including on how to fit facemasks Footnote 3 . In Hawai’i, the advice has been tailored to local community lifestyles and published on a dedicated “vog dashboard” Footnote 4 that is a single, freely accessible source of information, supported by multiple agencies. In multiple locations, ash, gas, and aerosol dispersion forecasts are linked to health information and advice for ongoing eruptions (Businger et al. 2015 ; Shiozawa et al. 2018 ; Barsotti et al. 2020 ). In Iceland, volcanic air pollution forecasts have been broadcast via radio and television and are available online (including social media) (Barsotti et al. 2020 ).
Emerging themes, knowledge gaps, and future directions
In general, few studies of health hazards and impacts are conducted relative to the number of eruptions that occur globally. Since 2001, the Global Volcanism Program Footnote 5 has reported 124 eruptions of VEI ≥3, while Table 2 reports 48 primary medical studies (at 23 volcanoes) assessing physical health effects of volcanic emissions. However, most of these studies were conducted in advanced-economy countries, notably the USA, Japan, and Iceland. Indonesia, with a 2021 population of ~277 million Footnote 6 and recent sustained and/or major eruptions of Merapi, Sinabung, Agung, and Semeru volcanoes, is notably under-represented, with a single clinical case study (Trisnawati et al. 2015 ). This inequality in attention, which relates to resources, opportunity, contacts, politics, and historical legacy, has meant that the health impacts of many major and sometimes long-duration eruptions near large populations have gone completely unstudied. Additionally, with a few exceptions (e.g., Kīlauea, Holuhraun, and Miyakejima) where multiple studies of the health effects of exposure to SO 2 and sulfate aerosol are reported (Table 2 ), effects of prolonged degassing have received little attention, relative to explosive eruptions, despite the chronic exposures and likely health effects.
A major research direction must be the development of methods for accurate exposure assessment. Further improvement of meteorological and dispersion models can help calculate ground-based pollutant concentrations at higher spatial and temporal resolution. Refining input parameters, plume models, and dynamic boundary layer representation, or incorporating advanced mathematical models such as Large Eddy Simulation, may also lead to much improved modeled concentrations (Barsotti et al. 2020 ; Burton et al. 2020 , Holland et al. 2020 ; Filippi et al. 2021 ). Limitations in the accuracy and speciation of ground-level concentrations from models or space-based instruments will require the continuation of ground-based in situ measurements. Installation of networks of low-cost gas and particulate sensors is becoming increasingly feasible with a proliferation of technology in the past decade Footnote 7 . Such networks provide exciting opportunities for collaborative science with local communities. However, there are challenges for deployment during crises in terms of procurement and delivery in humanitarian situations where agencies have other priorities, and transport and other critical infrastructure networks may be disrupted. Currently, the utility of low-cost sensors is much greater when they are benchmarked against reference-grade instruments, which may not be available, even regionally. Future improvements in sensor accuracy, calibration, and reliable global satellite internet may contribute to better exposure assessment (Kizel et al. 2018 ; Crawford et al. 2021 ).
We also foresee that air pollution research, in general, will move beyond a reliance on PM mass concentrations to assess impact and towards an understanding of the distinct PM chemical constituents, including metals and organic compounds, as well as towards physicochemical (e.g., surface area) or biological (e.g., oxidative potential) exposure metrics.
Interactions between volcanic eruptions and the ambient atmosphere and climate are an important future research direction with respect to health impacts. Ambient conditions influence the atmospheric dispersion and lifetime of volcanic emissions (for example, the sulfur gas-to-particle conversion rate; Gíslason et al. 2015 ), and ash remobilization in arid, windy climates may prolong population exposure (Jarvis et al. 2020 ). The consequences of global climate change for volcanic emission hazards are poorly understood but likely appreciable; for example, predicted weakening of Pacific trade winds will affect dispersion of emissions in Hawai’i and Vanuatu (Collins et al. 2010 ).
The greatest overall barrier to advancing our understanding of volcanic air pollution effects on human health is the scarcity of epidemiological and clinical studies. To facilitate future studies, and support risk management, especially where local syndromic surveillance is absent, standardized epidemiological protocols (Mueller et al. 2020b ) and crisis response resources Footnote 8 have recently been developed. Instigating such studies will be facilitated by continued co-development of standardized protocols, supporting local study teams and procuring equipment, funding, and ethical permissions. Relationship building between visiting researchers and host country academic, observatory, and agency partners is vital for preserving host countries’ intellectual property and ensuring beneficial research outcomes for impacted communities. In turn, this can support the effective communication of health impacts of volcanic air pollution to populations, health practitioners, and emergency managers.
https://uk-air.defra.gov.uk/networks/network-info?view=metals
www.ivhhn.org
https://www.ivhhn.org/information#printable
https://www.vog.ivhhn.org
https://volcano.si.edu/
https://www.worldometers.info/world-population/indonesia-population/
http://www.aqmd.gov/aq-spec
https://www.ivhhn.org/crisis-management
Allibone R, Cronin SJ, Charley DT, Neall VE, Stewart RB, Oppenheimer C (2012) Dental fluorosis linked to degassing of Ambrym volcano, Vanuatu: a novel exposure pathway. Environ Geochem Health 34:155–170. https://doi.org/10.1007/s10653-010-9338-2
Article Google Scholar
Amaral AF, Rodrigues AS (2007) Chronic exposure to volcanic environments and chronic bronchitis incidence in the Azores, Portugal. Environ Res 103:419–423. https://doi.org/10.1016/j.envres.2006.06.016
Armienta MA, De la Cruz-Reyna S, Morton O, Cruz O, Ceniceros N (2002) Chemical variations of tephra-fall deposit leachates for three eruptions from Popocatépetl volcano. Journal of Volcanology and Geothermal Research 113:61–80. https://doi.org/10.1016/S0377-0273(01)00251-7
Armienta MA, Cruz-Reyna S, Cruz O, Ceniceros N, Aguayo A, Marin M (2011) Fluoride in ash leachates: environmental implications at Popocatépetl volcano, central Mexico. Natural Hazards and Earth System Sciences 11:1949–1956. https://doi.org/10.5194/nhess-11-1949-2011
Atanga MBS, Van der Meerve AS, Shemamg EM, Suh CE, Kruger W, Njome MS, Asobo NE (2009) Volcanic Ash from the 1999 Eruption of Mount Cameroon volcano: characterization and implications to health hazards. Afr J Online 8:63–70. https://www.ajol.info/index.php/jcas/article/view/87049
Balsa AI, Caffera M, Bloomfield J (2016) Exposures to particulate matter from the eruptions of the Puyehue Volcano and birth outcomes in Montevideo, Uruguay. Environ Health Perspect 124:1816–1822. https://doi.org/10.1289/EHP235
Barone G, De Giudici G, Gimeno D, Lanzafame G, Podda F, Cannas C, Giuffrida A, Barchitta M, Agodi A, Mazzoleni P (2021) Surface reactivity of Etna volcanic ash and evaluation of health risks. Science of the Total Environment 761:143248. https://doi.org/10.1016/j.scitotenv.2020.143248
Barsotti S (2020) Probabilistic hazard maps for operational use: the case of SO 2 air pollution during the Holuhraun eruption (Bárðarbunga, Iceland) in 2014–2015. Bull Volcanol 82:56. https://doi.org/10.1007/s00445-020-01395-3
Barsotti S, Oddsson B, Gudmundsson MT, Pfeffer MA, Parks MM, Ófeigsson BG, Sigmundsson F, Reynisson V, Jónsdóttir K, Roberts MJ, Heiðarsson EP, Jónasdóttir EB, Einarsson P, Jóhannsson T, Gylfason ÁG, Vogfjörd K (2020) Operational response and hazards assessment during the 2014–2015 volcanic crisis at Bárðarbunga volcano and associated eruption at Holuhraun, Iceland. J Volcanol Geotherm Res 390:106753. https://doi.org/10.1016/j.jvolgeores.2019.106753
Bates MN, Garrett N, Crane J, Balmes JR (2013) Associations of ambient hydrogen sulfide exposure with self-reported asthma and asthma symptoms. Environ Res 122:81–87. https://doi.org/10.1016/j.envres.2013.02.002
Bates MN, Crane J, Balmes JR, Garrett N (2015) Investigation of hydrogen sulfide exposure and lung function, asthma and chronic obstructive pulmonary disease in a geothermal area of New Zealand. PLoS One 10:e0122062. https://doi.org/10.1371/journal.pone.0122062
Baxter PJ, Bonadonna C, Dupree R, Hards VL, Kohn SC, Murphy MD, Nichols A, Nicholson RA, Norton G, Searl A, Sparks RS, Vickers BP (1999) Cristobalite in volcanic ash of the Soufrière Hills volcano, Montserrat, British West Indies. Science 283:1142–1145. https://doi.org/10.1126/science.283.5405.1142
Baxter PJ, Searl AS, Cowie HA, Jarvis D, Horwell CJ (2014) Evaluating the respiratory health risks of volcanic ash at the eruption of the Soufrière Hills volcano, Montserrat, 1995-2010. Geological Society of London Memoirs 39:407–425. https://doi.org/10.1144/M39.22
BéruBé KA, Jones TP, Housley DG, Richards RJ (2004) The respiratory toxicity of airborne volcanic ash from the Soufrière Hills volcano, Montserrat. Mineralogical Magazine 68:47–60. https://doi.org/10.1180/0026461046810170
Bia G, Borgnino L, Zampieri G, Garcia MG (2020) Fluorine surface speciation in South Andean volcanic ashes. Chemical Geology 532:119402. https://doi.org/10.1016/j.chemgeo.2019.119402
Bosshard-Stadlin SA, Mattsson HB, Stewart C, Reusser E (2017) Leaching of lava and tephra from the Oldoinyo Lengai volcano (Tanzania): remobilization of fluorine and other potentially toxic elements into surface waters of the Gregory Rift. Journal of Volcanology and Geothermal Research 332:14–25. https://doi.org/10.1016/j.jvolgeores.2017.01.009
Brown SK, Jenkins SF, Sparks RSJ, Odbert H, Auker MR (2017) Volcanic fatalities database: analysis of volcanic threat with distance and victim classification. J Appl Volcanol 6:15. https://doi.org/10.1186/s13617-017-0067-4
Budianta W (2011) The potential impact of ash Merapi Volcano eruption 2010 in Yogyakarta, Indonesia, for the environment and human health. Journal of Applied Geology 3:111-115. https://doi.org/10.22146/jag.7187
Google Scholar
Burton RR, Woodhouse MJ, Gadian AM, Mobbs SD (2020) The use of a numerical weather prediction model to simulate near-field volcanic plumes. Atmosphere 11:594. https://doi.org/10.3390/atmos11060594
Businger S, Huff R, Horton K, Sutton AJ, Elias T (2015) Observing and forecasting vog dispersion from Kīlauea Volcano, Hawai’i. Bull Amer Meteor Soc 96:1667–1686. https://doi.org/10.1175/BAMS-D-14-00150.1
Cadelis G, Tourres R, Molinie J, Petit RH (2013) Exacerbations of asthma in Guadeloupe (French West Indies) and volcanic eruption in Montserrat (70 km from Guadeloupe). Rev Mal Respir 30:203–214. https://doi.org/10.1016/j.rmr.2012.11.002
Camara JG, Lagunzad JK (2011) Ocular findings in volcanic fog induced conjunctivitis. Hawai’i Med J 70:262–265
Cangemi M, Speziale S, Madonia P, D'Alessandro W, Andronico D, Bellomo S, Brusca L, Kyriakopoulos K (2017) Potentially harmful elements released by volcanic ashes: examples from the Mediterranean area. Journal of Volcanology and Geothermal Research 337:16–28. https://doi.org/10.1016/j.jvolgeores.2017.03.015
Carlsen HK, Gíslason T, Benediktsdóttir B, Kolbeinsson TB, Hauksdóttir A, Thorsteinsson T, Briem H (2012a) A survey of early health effects of the Eyjafjallajökull 2010 eruption in Iceland: a population-based study. BMJ Open 2:e000343. https://doi.org/10.1136/bmjopen-2011-000343
Carlsen HK, Hauksdóttir A, Valdimarsdóttir UA, Gíslason T, Einarsdóttir G, Runolfsson H, Briem H, Finnbjornsdóttir RG, Gudmundsson S, Kolbeinsson TB, Thorsteinsson T, Pétursdóttir G (2012b) Health effects following the Eyjafjallajökull volcanic eruption: a cohort study. BMJ Open 2:e001851. https://doi.org/10.1136/bmjopen-2012-001851
Carlsen HK, Aspelund T, Briem H, Gíslason T, Jóhannsson T, Valdimarsdóttir U, Gudnason T (2019) Respiratory health among professionals exposed to extreme SO 2 levels from a volcanic eruption. Scand J Work Environ Health 45:312–315. https://doi.org/10.5271/sjweh.3783
Carlsen HK, Ilyinskaya E, Baxter PJ, Schmidt A, Thorsteinsson T, Pfeffer MA, Barsotti S, Dominici F, Finnbjornsdóttir RG, Jóhannsson T, Aspelund T, Gíslason T, Valdimarsdóttir U, Briem H, Gudnason T (2021a) Increased respiratory morbidity associated with exposure to a mature volcanic plume from a large Icelandic fissure eruption. Nat Commun 12:2161. https://doi.org/10.1038/s41467-021-22432-5
Carlsen HK, Valdimarsdóttir U, Briem H et al (2021b) Severe volcanic SO 2 exposure and respiratory morbidity in the Icelandic population – a register study. Environ Health 20:23. https://doi.org/10.1186/s12940-021-00698-y
Chow DC, Grandinetti A, Fernandez E, Sutton AJ, Elias T, Brooks B, Tam EK (2010) Is volcanic air pollution associated with decreased heart-rate variability? Heart Asia 2:36–41. https://doi.org/10.1136/ha.2009.001172
Collins M, An SI, Cai W et al (2010) The impact of global warming on the tropical Pacific Ocean and El Niño. Nature Geosci 3:391–397. https://doi.org/10.1038/ngeo868
Connell J, Lutkehaus N (2017) Escaping Zaria’s fire? The volcano resettlement problem of Manam Island, Papua New Guinea. Asia Pacific Viewpoint 58:14. https://doi.org/10.1111/apv.12148
Crawford B, Hagan DH, Grossman I, Cole E, Holland L, Heald CL, Kroll JH (2021) Mapping pollution exposure and chemistry during an extreme air quality event (the 2018 Kilauea eruption) using a low-cost sensor network. PNAS 118:27. https://doi.org/10.1073/pnas.2025540118
Cronin SJ, Sharp DS (2002) Environmental impacts on health from continuous volcanic activity at Yasur (Tanna) and Ambrym, Vanuatu. International Journal of Environmental Health Research 12:109–123. https://doi.org/10.1080/09603120220129274
Cronin SJ, Stewart C, Zernack AV, Brenna M, Procter JN, Pardo N, Christenson B, Wilson T, Stewart RB, Irwin M (2014) Volcanic ash leachate compositions and assessment of health and agricultural hazards from 2012 hydrothermal eruptions, Tongariro, New Zealand. Journal of Volcanology and Geothermal Research 286:233–247. https://doi.org/10.1016/j.jvolgeores.2014.07.002
Cullen RT, Jones AD, Miller BG, Tran CL, Davis JM, Donaldson K, Wilson M, Stone V, Morgan A (2002) Toxicity of volcanic ash from Montserrat. Institute of Occupational Medicine Research Report TM/02/01 April 2002. https://citeseerx.ist.psu.edu/viewdoc/download?doi=10.1.1.599.9295&rep=rep1&type=pdf
Cuthbertson J, Stewart C, Lyon A, Burns P, Telopo T (2020) Health impacts of volcanic activity in Oceania. Prehospital and Disaster Medicine 35:574–578. https://doi.org/10.1017/S1049023X2000093X
D’Alessandro W (2006) Human fluorosis related to volcanic activity: a review. In: WIT Transactions on Biomedicine and Health 10:21–30. https://doi.org/10.2495/ETOX060031
Daga R, Guevara SR, Poire DG, Arribére M (2014) Characterization of tephras dispersed by the recent eruptions of volcanoes Calbuco (1961), Chaitén (2008) and Cordón Caulle Complex (1960 and 2011), in Northern Patagonia. Journal of South American Earth Sciences 49:1–4. https://doi.org/10.1016/j.jsames.2013.10.006
Damby DE (2012) From dome to disease: The respiratory toxicity of volcanic cristobalite. Dissertation, Durham University. http://etheses.dur.ac.uk/7328/1/damby_thesis.pdf
Damby DE, Horwell CJ, Baxter PJ, Delmelle P, Donaldson K, Dunster C, Fubini B, Murphy FA, Nattrass C, Sweeney S, Tetley TD (2013) The respiratory health hazard of tephra from the 2010 Centennial eruption of Merapi with implications for occupational mining of deposits. Journal of Volcanology and Geothermal Research 261:376–387. https://doi.org/10.1016/j.jvolgeores.2012.09.001
Damby DE, Llewellin EW, Horwell CJ, Williamson BJ et al (2014) The alpha-beta phase transition in volcanic cristobalite. J Appl Crystallogr 47:4. https://doi.org/10.1107/S160057671401070X
Damby DE, Murphy FA, Horwell CJ, Raftis J, Donaldson K (2016) The in vitro respiratory toxicity of cristobalite-bearing volcanic ash. Environ Res 145:74–84. https://doi.org/10.1016/j.envres.2015.11.020
Damby DE, Horwell CJ, Larsen G, Thordarson T, Tomatis M, Fubini B, Donaldson K (2017) Assessment of the potential respiratory hazard of volcanic ash from future Icelandic eruptions: a study of archived basaltic to rhyolitic ash samples. Environ Health 16:98. https://doi.org/10.1186/s12940-017-0302-9
Damby DE, Peek S, Lerner AH, Elias T (2018a) Volcanic ash leachate chemistry from increased 2018 activity of Kīlauea volcano. U.S. Geological Survey data release, Hawai’i. https://doi.org/10.5066/P98A07DC . https://www.sciencebase.gov/catalog/item/5b69cbe6e4b006a11f775784
Damby DE, Horwell CJ, Baxter PJ, Kueppers U et al (2018b) Volcanic ash activates the NLRP3 inflammasome in murine and human macrophages. Front Immunol 8:2000. https://doi.org/10.3389/fimmu.2017.02000
de Lima EF, Sommer CA, Cordeiro Silva IM, Netta AP, Lindenberg M, Marques Alves R (2012) Morphology and chemistry of the Puyehue volcano ashes deposited at Porto Alegre metropolitan region in June 2011. Revista Brasiliera de Geociências 42:265–280. https://doi.org/10.5327/Z0375-75362012000200004
Delmelle P (2003) Environmental impacts of tropospheric volcanic gas plumes. Geol Soc Lond Spec Publ 213:381–399. https://doi.org/10.1144/GSL.SP.2003.213.01.23
Donaldson K, Borm PJA (1998) The quartz hazard: a variable entity. The Annals of Occupational Hygiene 42:287–294. https://doi.org/10.1093/annhyg/42.5.287
Doocy S, Daniels A, Dooling S, Gorokhovich Y (2013) The human impact of volcanoes: a historical review of events 1900-2009 and systematic literature review. PLoS Curr 16:5. https://doi.org/10.1371/currents.dis.841859091a706efebf8a30f4ed7a1901
Durand M, Wilson JG (2006) Spatial analysis of respiratory disease on an urbanized geothermal field. Environ Res 101:238–245. https://doi.org/10.1016/j.envres.2005.08.006
Durant AJ, Villarosa G, Rose WI, Delmelle P, Prata AJ, Viramonte JG (2012) Long-range volcanic ash transport and fallout during the 2008 eruption of Chaitén volcano, Chile. Physics and Chemistry of the Earth Parts A/B/C 45–46:50–64. https://doi.org/10.1016/j.pce.2011.09.004
Elliot AJ, Singh N, Loveridge P, Harcourt S, Smith S, Pnaiser R, Kavanagh K, Robertson C, Ramsay CN, McMenamin J, Kibble A, Murray V, Ibbotson S, Catchpole M, McCloskey B, Smith GE (2010) Syndromic surveillance to assess the potential public health impact of the Icelandic volcanic ash plume across the United Kingdom, April 2010. Euro Surveill 15:19583
Eychenne J, Cashman K, Rust A, Durant A (2015) Impact of the lateral blast on the spatial pattern and grain size characteristics of the 18 May 1980 Mount St. Helens fallout deposit. J Geophys Res Solid Earth 120:6018–6038. https://doi.org/10.1002/2015JB012116
Fano V, Cernigliaro A, Scondotto S, Perucci CA, Forastiere F (2010) The fear of volcano: short-term health effects after Mount Etna's eruption in 2002. Eur Respir J 36:1216–1218. https://doi.org/10.1183/09031936.00078910
Felton D, Grange G, Damby DE, Bronstein A, Spyker D (2019) Sulfur dioxide monitoring associated with the 2018 Kilauea Lower East Rift Zone Eruption. International Union of Toxicology (IUTOX) 15th International Congress of Toxicology, Honolulu, HI, USA
Filippi J-B, Durand J, Tulet P, Bielli S (2021) Multiscale modeling of convection and pollutant transport associated with volcanic eruption and lava flow: application to the April 2007 eruption of the Piton de la Fournaise (Réunion Island). Atmosphere 12:507. https://doi.org/10.3390/atmos12040507
Forbes L, Jarvis D, Potts J, Baxter PJ (2003) Volcanic ash and respiratory symptoms in children on the island of Montserrat, British West Indies. Occup Environ Med 60:207–211. https://doi.org/10.1136/oem.60.3.207
Freire S, Florczyk AJ, Pesaresi M, Sliuzas R (2019) An improved global analysis of population distribution in proximity to active volcanoes, 1975–2015. ISPRS International Journal of Geo-Information 8:341. https://doi.org/10.3390/ijgi8080341
Gíslason SR, Stefánsdóttir G, Pfeffer MA, Barsotti S, Jóhannsson Th, Galeczka I, Bali E, Sigmarsson O, Stefánsson A, Keller NS, Sigurdsson Á, Bergsson B, Galle B, Jacobo VC, Arellano S, Aiuppa A, Jónasdóttir EB, Eiríksdóttir ES, Jakobsson S, Guðfinnsson GH, Halldórsson SA, Gunnarsson H, Haddadi B, Jónsdóttir I, Thordarson Th, Riishuus M, Högnadóttir Th, Dürig T, Pedersen GBM, Höskuldsson Á, Gudmundsson MT (2015) Environmental pressure from the 2014-15 eruption of Bárðarbunga volcano, Iceland. Geochemical Perspectives Letters 1:84-93. https://doi.org/10.7185/geochemlet.1509
Greenberg MI, Waksman J, Curtis J (2007) Silicosis: a review. Disease-a-Month 53:394–416. https://doi.org/10.1016/j.disamonth.2007.09.020
Gudmundsson G (2011) Respiratory health effects of volcanic ash with special reference to Iceland: a review. Clin Respir J 5:2–9. https://doi.org/10.1111/j.1752-699X.2010.00231.x
Hansell A, Oppenheimer C (2004) Health hazards from volcanic gases: a systematic literature review. Arch Environ Health 59:628–639. https://doi.org/10.1080/00039890409602947
Heaviside C, Witham C, Vardoulakis S (2021) Potential health impacts from sulfur dioxide and sulfate exposure in the UK resulting from an Icelandic effusive eruption. Science of the Total Environment 774:145549. https://doi.org/10.1016/j.scitotenv.2021.145549
Heggie TW (2005) Reported fatal and non-fatal incidents involving tourists in Hawai’i Volcanoes National Park, 1992-2002. Travel Med Infect Dis 3:123–131. https://doi.org/10.1016/j.tmaid.2004.09.004
Heggie TW, Heggie TM, Heggie TJ (2009) Death by volcanic laze. Wilderness Environ Med 20:101–103. https://doi.org/10.1580/08-WEME-LE-236.1
Higuchi K, Koriyama C, Akiba S (2012) Increased mortality of respiratory diseases, including lung cancer, in the area with large amount of ashfall from Mount Sakurajima volcano. J Environ Public Health 2012:257831. https://doi.org/10.1155/2012/257831
Hillman SE, Horwell CJ, Densmore AL, Damby DE, Fubini B, Ishimine Y, Tomatis M (2012) Sakurajima volcano: a physico-chemical study of the health consequences of long-term exposure to volcanic ash. Bulletin of Volcanology 74:913–930. https://doi.org/10.1007/s00445-012-0575-3
Hincks TK, Aspinall WP, Baxter PJ, Searl A, Sparks RSJ, Woo G (2006) Long-term exposure to respirable volcanic ash on Montserrat: a time-series simulation. Bull Volcanol 68:264–266. https://doi.org/10.1007/s00445-005-0006-9
Hlodversdóttir H, Petursdóttir G, Carlsen HK, Gíslason T, Hauksdóttir A (2016) Long-term health effects of the Eyjafjallajökull volcanic eruption: a prospective cohort study in 2010 and 2013. BMJ Open 6(9):e011444. https://doi.org/10.1136/bmjopen-2016-011444
Hlodversdóttir H, Thorsteinsdóttir H, Thordardóttir EB, Njardvik U, Petursdóttir G, Hauksdóttir A (2018) Long-term health of children following the Eyjafjallajökull volcanic eruption: a prospective cohort study. Eur J Psychotraumatol 9:1442601. https://doi.org/10.1080/20008198.2018.1442601
Holland L, Businger S, Elias T, Cherubini T (2020) Two ensemble approaches for forecasting sulfur dioxide concentrations from Kīlauea Volcano. Wea Forecasting 35:1923–1937. https://doi.org/10.1175/WAF-D-19-0189.1
Horwell CJ (2007) Grain-size analysis of volcanic ash for the rapid assessment of respiratory health hazard. Journal of Environmental Monitoring 9:1107–1115. https://doi.org/10.1039/B710583P
Horwell CJ, Baxter PJ (2006) The respiratory health hazards of volcanic ash: a review for volcanic risk mitigation. Bulletin of Volcanology 69:1–24. https://doi.org/10.1007/s00445-006-0052-y
Horwell CJ, Sparks RSJ, Brewer TS, Llewellin EW, Williamson BJ (2003a) Characterization of respirable volcanic ash from the Soufrière Hills volcano, Montserrat, with implications for human health hazards. Bulletin of Volcanology 65:346–362. https://doi.org/10.1007/s00445-002-0266-6
Horwell CJ, Fenoglio I, Ragnarsdóttir KV, Sparks RSJ, Fubini B (2003b) Surface reactivity of volcanic ash from the eruption of Soufrière Hills volcano, Montserrat, West Indies with implications for health hazards. Environmental Research 93:202–215. https://doi.org/10.1016/S0013-9351(03)00044-6
Horwell CJ, Fenoglio I, Fubini B (2007) Iron-induced hydroxyl radical generation from basaltic volcanic ash. Earth Planet Sci Lett 261:662–669. https://doi.org/10.1016/j.epsl.2007.07.032
Horwell CJ, Le Blond JS, Michnowicz SA, Cressey G (2010a) Cristobalite in a rhyolitic lava dome: evolution of ash hazard. Bulletin of Volcanology 72:249–253. https://doi.org/10.1007/s00445-009-0327-1
Horwell CJ, Stannett GW, Andronico D, Bertagnini A, Fenoglio I, Fubini B, Le Blond JS, Williamson BJ (2010b) A physico-chemical assessment of the health hazard of Mt. Vesuvius volcanic ash. J Volcanol Geotherm Res 191:222–232. https://doi.org/10.1016/j.jvolgeores.2010.01.014
Horwell C, Williamson B, Donaldson K, Le Blond J, Damby D, Bowen L (2012) The structure of volcanic cristobalite in relation to its toxicity; relevance for the variable crystalline silica hazard. Part Fibre Toxicol 9:44. https://doi.org/10.1186/1743-8977-9-44
Horwell CJ, Baxter PJ, Hillman SE, Calkins JA, Damby DE, Delmelle P, Donaldson K, Dunster C, Fubini B, Kelly FJ, Le Blond JS (2013) Physicochemical and toxicological profiling of ash from the 2010 and 2011 eruptions of Eyjafjallajökull and Grímsvötn volcanoes, Iceland using a rapid respiratory hazard assessment protocol. Environmental Research 127:63–73. https://doi.org/10.1016/j.envres.2013.08.011
Horwell CJ, Hillman SE, Cole, PD, Loughlin SC, Llewellin EW, Damby DE, Christopher TE (2014) Controls on variations in cristobalite abundance in ash generated by the Soufrière Hills Volcano, Montserrat in the period 1997 to 2010. In: Wadge G, Robertson REA and Voight B (eds .) The eruption of Soufrière Hills Volcano, Montserrat from 2000 to 2010. Geological Society of London 399-406. (Memoir 39). https://doi.org/10.1144/M39.21
Horwell CJ, Sargent P, Andronico D, Castro ML, Tomatis M, Hillman SE, Michnowicz SA, Fubini B (2017) The iron-catalysed surface reactivity and health-pertinent physical characteristics of explosive volcanic ash from Mt. Etna. Italy. Journal of Applied Volcanology 6:1–6. https://doi.org/10.1186/s13617-017-0063-8
Horwell CJ, Covey J, Merli C, Dominelli et al (2020) Preparing for, and protecting communities from, respiratory exposure to volcanic ash. PAHO/WHO Emergencies Disasters Newsletter, Supplement on Health Disaster Risk Reduction. October 2020, Issue 130. https://www3.paho.org/disasters/newsletter/index.php?option=com_content&view=category&id=320&Itemid=101&lang=en
Ilyinskaya E, Schmidt A, Mather TA, Pope FD, Witham C, Baxter P, Jóhannsson T, Pfeffer M, Barsotti S, Singh A, Sanderson P, Bergsson B, Kilbride BM, Donovan A, Peters N, Oppenheimer C, Edmonds M (2017) Understanding the environmental impacts of large fissure eruptions: aerosol and gas emissions from the 2014–2015 Holuhraun eruption (Iceland). Earth Planet Sci Lett 472:309–322. https://doi.org/10.1016/j.epsl.2017.05.025
Ilyinskaya E, Mason E, Wieser PE et al (2021) Rapid metal pollutant deposition from the volcanic plume of Kīlauea, Hawai’i. Commun Earth Environ 2:78. https://doi.org/10.1038/s43247-021-00146-2
International Agency for Research on Cancer (1997) Silica, some silicates, coal dust and para-aramid fibrils. IARC Monographs on the Evaluation of Carcinogenic Risks to Humans 68
Ishigami A, Kikuchi Y, Iwasawa S, Nishiwaki Y, Takebayashi T, Tanaka S, Omae K (2008) Volcanic sulfur dioxide and acute respiratory symptoms on Miyakejima island. Occup Environ Med 65:701–707. https://doi.org/10.1136/oem.2007.033456
Iwasawa S, Kikuchi Y, Nishiwaki Y, Nakano M, Michikawa T, Tsuboi T, Tanaka S, Uemura T, Ishigami A, Nakashima H, Takebayashi T, Adachi M, Morikawa A, Maruyama K, Kudo S, Uchiyama I, Omae K (2009) Effects of SO 2 on respiratory system of adult Miyakejima residents 2 years after returning to the island. J Occup Health 51:38–47. https://doi.org/10.1539/joh.l8075
Iwasawa S, Nakano M, Tsuboi T, Kochi T, Tanaka S, Katsunuma T, Morikawa A, Omae K (2015) Effects of sulfur dioxide on the respiratory system of Miyakejima child residents 6 years after returning to the island. Int Arch Occup Environ Health 88:1111–1118. https://doi.org/10.1007/s00420-015-1037-y
Jarvis P, Bonadonna C, Dominguez L et al (2020) Aeolian remobilisation of volcanic ash: outcomes of a workshop in the Argentinian Patagonia. Frontiers in Earth Sciences 8. https://doi.org/10.3389/feart.2020.575184
Jenkins SF, Wilson T, Magill C, Miller V, Stewart C, Blong R, Marzocchi W, Boulton M, Bonadonna C, Costa A (2015) Volcanic ash fall hazard and risk. In: Loughlin SC, Sparks RSJ, Brown SK, Jenkins SF, Vye-Brown C (eds) Global Volcanic Hazards and Risk. Cambridge University Press, Cambridge. https://doi.org/10.1017/CBO9781316276273.005
Jones T, BéruBé K (2011) The bioreactivity of the sub-10 μm component of volcanic ash: Soufrière Hills volcano, Montserrat. Journal of Hazardous Materials 194:128–134. https://doi.org/10.1016/j.jhazmat.2011.07.092
Kelly FJ (2003) Oxidative stress: its role in air pollution and adverse health effects. Occup Environ Med 60:612–616. https://doi.org/10.1136/oem.60.8.612
Kimura K, Sakamoto T, Miyazaki M, Uchino E, Kinukawa N, Isashiki M (2005) Effects of volcanic ash on ocular symptoms: results of a 10-year survey on schoolchildren. Ophthalmology 112:478–481. https://doi.org/10.1016/j.ophtha.2004.09.031
Kizel F, Etzion Y, Shafran-Nathan R, Levy I, Fishbain B, Bartonova A, Broday DM (2018) Node-to-node field calibration of wireless distributed air pollution sensor network. Environmental Pollution 233:900–909. https://doi.org/10.1016/j.envpol.2017.09.042
Kochi T, Iwasawa S, Nakano M, Tsuboi T, Tanaka S, Kitamura H, Wilson DJ, Takebayashi T, Omae K (2017) Influence of sulfur dioxide on the respiratory system of Miyakejima adult residents 6 years after returning to the island. J Occup Health 59:313–326. https://doi.org/10.1539/joh.16-0256-OA
Le Blond JS, Cressey G, Horwell CJ, Williamson BJ (2009) A rapid method for quantifying single mineral phases in heterogeneous natural dusts using X-ray diffraction. Powder Diffract 24:17–23. https://doi.org/10.1154/1.3077941
Le Blond JS, Horwell CJ, Baxter PJ, Michnowicz SA, Tomatis M, Fubini B, Delmelle P, Dunster C, Patia H (2010) Mineralogical analyses and in vitro screening tests for the rapid evaluation of the health hazard of volcanic ash at Rabaul volcano, Papua New Guinea. Bulletin of Volcanology 72:1077–1092. https://doi.org/10.1007/s00445-010-0382-7
Lee SH, Richards RJ (2004) Montserrat volcanic ash induces lymph node granuloma and delayed lung inflammation. Toxicology 195:155–165. https://doi.org/10.1154/1.3077941
Linhares D, Ventura Garcia P, Viveiros F, Ferreira T, dos Santos RA (2015) Air pollution by hydrothermal volcanism and human pulmonary function. Biomed Res Int 2015:326794. https://doi.org/10.1155/2015/326794
Lombardo D, Ciancio N, Campisi R, Di Maria A, Bivona L, Poletti V, Mistretta A, Biggeri A, Di Maria G (2013) A retrospective study on acute health effects due to volcanic ash exposure during the eruption of Mount Etna (Sicily) in 2002. Multidiscip Respir Med 8:51. https://doi.org/10.1186/2049-6958-8-51
Longo BM (2009) The Kilauea Volcano adult health study. Nurs Res 58:23–31. https://doi.org/10.1097/NNR.0b013e3181900cc5
Longo BM (2013) Adverse health effects associated with increased activity at Kīlauea Volcano: a repeated population-based survey. ISRN Public Health 1-10. https://doi.org/10.1155/2013/475962
Longo BM, Yang W (2008) Acute bronchitis and volcanic air pollution: a community-based cohort study at Kilauea Volcano, Hawai'i, USA. J Toxicol Environ Health A 71:1565–1571. https://doi.org/10.1080/15287390802414117
Longo BM, Rossignol A, Green JB (2008) Cardiorespiratory health effects associated with sulphurous volcanic air pollution. Public Health 122:809–820. https://doi.org/10.1016/j.puhe.2007.09.017
Longo BM, Yang W, Green JB, Crosby FL, Crosby VL (2010) Acute health effects associated with exposure to volcanic air pollution (vog) from increased activity at Kilauea Volcano in 2008. J Toxicol Environ Health A 73:1370–1381. https://doi.org/10.1080/15287394.2010.497440
Macomber P (2020) Guidelines on rainwater catchment systems for Hawai’i. College of Tropical Agriculture and Human Resources, University of Hawai’i. https://www.ctahr.hawaii.edu/hawaiirain/Library/Guides&Manuals/HI_Guidelines_2020.pdf
Mason E, Wieser PE, Liu EJ, Edmonds M, Ilyinskaya E, Whitty RCW, Mather TA, Elias T, Nadeau PA, Wilkes TC, McGonigle AJS, Pering TD, Mims FM, Kern C, Schneider D, Oppenhemier C (2021) Volatile metal emissions from volcanic degassing and lava–seawater interactions at Kīlauea Volcano. Hawai’i. Commun Earth Environ 2:79. https://doi.org/10.1038/s43247-021-00145-3
Mather TA, Allen AG, Oppenheimer C et al (2003) Size-resolved characterisation of soluble ions in the particles in the tropospheric plume of Masaya Volcano, Nicaragua: Origins and Plume Processing. J Atmos Chem 46:207–237. https://doi.org/10.1023/A:1026327502060
McDonald F, Horwell CJ (2021) Air pollution disasters: Liability issues in negligence associated with the provision of personal protective interventions (facemasks). Disaster Medicine and Public Health Preparedness 15:367–373. https://doi.org/10.1017/dmp.2020.37
McDonald F, Horwell CJ, Wecker R, Dominelli L, Loh M, Kamanyire R, Ugarte C (2020) Facemask use for community protection from air pollution disasters: an ethical overview and framework to guide agency decision making. International Journal of Disaster Risk Reduction 43:101376. https://doi.org/10.1016/j.ijdrr.2019.101376
Michaud JP, Grove JS, Krupitsky D (2004) Emergency department visits and “vog”-related air quality in Hilo, Hawai'i. Environ Res 95:11–19. https://doi.org/10.1016/S0013-9351(03)00122-1
Michellier C, Katoto PMC, Dramaix M, Nemery B, Kervyn F (2020) Respiratory health and eruptions of the Nyiragongo and Nyamulagira volcanoes in the Democratic Republic of Congo: a time-series analysis. Environ Health 9:62. https://doi.org/10.1186/s12940-020-00615-9
Monick MM, Baltrusaitis J, Powers LS, Borcherding JA, Caraballo JC, Mudunkotuwa I, Peate DW, Walters K, Thompson JM, Grassian VH, Gudmundsson G (2013) Effects of Eyjafjallajökull volcanic ash on innate immune system responses and bacterial growth in vitro. Environmental Health Perspectives 121:691–698. https://doi.org/10.1289/ehp.1206004
Mueller W, Horwell CJ, Apsley A, Steinle S, McPherson S, Cherrie JW, Galea KS (2018) The effectiveness of respiratory protection worn by communities to protect from volcanic ash inhalation. Part I: Filtration efficiency tests. Int J Hyg Environ Health 221:967–976. https://doi.org/10.1016/j.ijheh.2018.03.012
Mueller W, Cowie H, Horwell CJ, Hurley F, Baxter P (2020a) Health impact assessment of volcanic ash inhalation: a comparison with outdoor air pollution methods. GeoHealth 4:e2020GH000256. https://doi.org/10.1029/2020GH000256
Mueller W, Cowie H, Horwell CJ, Baxter PJ, McElvenny D, Booth M, Cherrie JW, Cullinan P, Jarvis D, Ugarte C, Inoue H (2020b) Standardised epidemiological protocols for populations affected by volcanic eruptions. Bull World Health Organ 98:362–364. https://doi.org/10.2471/BLT.19.244509
Nattrass C, Horwell CJ, Damby DE, Brown D, Stone V (2017) The effect of aluminium and sodium impurities on the in vitro toxicity and pro-inflammatory potential of cristobalite. Environ Res 159:164–175. https://doi.org/10.1016/j.envres.2017.07.054
Naumova EN, Yepes H, Griffiths JK, Sempértegui F, Khurana G, Jagai JS, Játiva E, Estrella B (2007) Emergency room visits for respiratory conditions in children increased after Guagua Pichincha volcanic eruptions in April 2000 in Quito, Ecuador observational study: time series analysis. Environ Health 6:21. https://doi.org/10.1186/1476-069X-6-21
Newnham RM, Dirks KN, Samaranayake D (2010) An investigation into long-distance health impacts of the 1996 eruption of Mt Ruapehu, New Zealand. Atmospheric Environment 44:1568–1578. https://doi.org/10.1016/j.atmosenv.2009.12.040
Nieto-Torres A, Martin-Del Pozzo AL (2021) Ash emission from a long-lived eruption at Popocatépetl volcano and mapped respiratory effects. Bulletin of Volcanology 83:68. https://doi.org/10.1007/s00445-021-01490-z
Olsson J, Stipp SL, Dalby KN, Gíslason SR (2013) Rapid release of metal salts and nutrients from the 2011 Grímsvötn, Iceland volcanic ash. Geochimica et Cosmochimica Acta 123:134–149. https://doi.org/10.1016/j.gca.2013.09.009
Oppenheimer C, Pyle DM, Barclay J (2003) Volcanic Degassing. Geological Society of London, Special Publications 213. https://doi.org/10.1144/GSL.SP.2003.213
Oudin A, Carlsen HK, Forsberg B, Johansson C (2013) Volcanic ash and daily mortality in Sweden after the Icelandic volcano eruption of May 2011. Int J Environ Res Public Health 10:6909–6919. https://doi.org/10.3390/ijerph10126909
Paez PA, Cogliati MG, Caselli AT, Monasterio AM (2021) An analysis of volcanic SO 2 and ash emissions from Copahue volcano. Journal of South American Earth Sciences 103365. https://doi.org/10.1016/j.jsames.2021.103365
Prüss-Ustün A, Vickers C, Haefliger P, Bertollini R (2011) Knowns and unknowns on burden of disease due to chemicals: a systematic review. Environ Health 10:9. https://doi.org/10.1186/1476-069X-10-9
Reich M, Zúñiga A, Amigo A, Vargas G, Morata D, Palacios C, Parada MA, Garreaud RD (2009) Formation of cristobalite nanofibers during explosive volcanic eruptions. Geology 37:435–438. https://doi.org/10.1130/G25457A.1
Rojas-Ramos M, Catalan-Vazquez M, Pozzo AL, Garcia-Ojeda E, Villalba-Caloca J, Perez-Neria J (2001) A seven month prospective study of the respiratory effects of exposure to ash from Popocatepetl volcano, Mexico. Environ Geochem Health 23:383–396. https://doi.org/10.1023/A:1012244311557
Schmidt A, Ostro B, Carslaw KS, Wilson M, Thordarson T, Mann GW, Simmons AJ (2011) Excess mortality in Europe following a future Laki-style Icelandic eruption. Proceedings of the National Academy of Sciences 108:15710–15715. https://doi.org/10.1073/pnas.1108569108
Schmidt A, Leadbetter S, Theys N et al. (2015) Satellite detection, long-range transport and air quality impacts of volcanic sulfur dioxide from the 2014–15 flood lava eruption at Bárðarbunga (Iceland). J Geophys Res Atmos JD023638. https://doi.org/10.1002/2015JD023638
Shimizu Y, Dobashi K, Hisada T, Ono A, Todokoro M, Iijima H, Utsugi M, Kakegawa S, Iizuka K, Ishizuka T, Morikawa A, Mori M (2007) Acute impact of volcanic ash on asthma symptoms and treatment. Int J Immunopathol Pharmacol 20:9–14. https://doi.org/10.1177/03946320070200S203
Shiozawa M, Lefor AK, Sata N, Yasuda Y, Nagai H (2018) Effects of the Miyakejima volcano eruption on public health. Int J Crit Care Emerg Med 4:040. https://doi.org/10.23937/2474-3674/1510040
Shojima J, Ikushima S, Ando T, Mochida A, Yanagawa T, Takemura T, Oritsu M (2006) A case of volcanic ash lung: report of a case. Nihon Kokyuki Gakkai Zasshi 44:192–196
Steinle S, Sleeuwenhoek A, Mueller W, Horwell CJ, Apsley A, Davis A, Cherrie JW, Galea KS (2018) The effectiveness of respiratory protection worn by communities to protect from volcanic ash inhalation. Part II: Total inward leakage tests. Int J Hyg Environ Health 221:977–984. https://doi.org/10.1016/j.ijheh.2018.03.011
Stewart C, Johnston DM, Leonard GS, Horwell CJ et al (2006) Contamination of water supplies by volcanic ashfall: a literature review and simple impact modelling. J Volcanol Geotherm Res 158:296–306. https://doi.org/10.1016/j.jvolgeores.2006.07.002
Stewart C, Cronin SJ, Wilson TM, Clegg S (2014) Analysis of Mt Sinabung volcanic ash: implications for animal health. GNS Science Report 2014/65; Available as free download from http://shop.gns.cri.nz/sr_2014-065-pdf/
Stewart C, Damby DE, Tomašek I, Horwell CJ, Plumlee G, Armienta MA, Ruiz Hinojosa MG, Appleby M, Delmelle P, Cronin S, Ottley CJ, Oppenhemier C, Morman S (2020) Assessment of leachable elements in volcanic ashfall: a review and evaluation of a standardized protocol for ash hazard characterization. Journal of Volcanology and Geothermal Research 392:106756. https://doi.org/10.1016/j.jvolgeores.2019.106756
Stewart C, Rosenberg MD, Kilgour GN (2021) Ash leachate characteristics of the 27 April 2016 and 13 September 2016 eruptions of Whakaari / White Island volcano. Lower Hutt (NZ): GNS Science. 15 p. (GNS Science report; 2020/39)
Tam E, Miike R, Labrenz S, Sutton AJ, Elias T, Davis J, Chen Y-L, Tantisira K, Dockery D, Avol E (2016) Volcanic air pollution over the Island of Hawai'i: emissions, dispersal, and composition. Association with respiratory symptoms and lung function in Hawai'i Island school children. Environment International 92-93:543–552. https://doi.org/10.1016/j.envint.2016.03.025
Tesone AI, Vitar RML, Tau J, Maglione GA, Llesuy S, Tasat DR, Berra A (2018) Volcanic ash from Puyehue-Cordón Caulle Volcanic Complex and Calbuco promote a differential response of pro-inflammatory and oxidative stress mediators on human conjunctival epithelial cells. Environmental Research 167:87–97. https://doi.org/10.1016/j.envres.2018.07.013
Tomašek I, Horwell CJ, Damby DE, Barošová H, Geers C, Petri-Fink A, Rothen-Rutishauser B, Clift MJD (2016) Combined exposure of diesel exhaust particles and respirable Soufrière Hills volcanic ash causes a (pro-)inflammatory response in an in vitro multicellular epithelial tissue barrier model. Part Fibre Toxicol 13:67. https://doi.org/10.1186/s12989-016-0178-9
Tomašek I, Horwell CJ, Bisig C, Damby DE, Comte P, Czerwinski J, Petri-Fink A, Clift MJD, Drasler B, Rothen-Rutishauser B (2018) Respiratory hazard assessment of combined exposure to complete gasoline exhaust and respirable volcanic ash in a multicellular human lung model at the air-liquid interface. Environ Pollut 238:977–987. https://doi.org/10.1016/j.envpol.2018.01.115
Tomašek I, Damby DE, Horwell CJ, Ayris PM, Delmelle P, Ottley CJ, Cubillas P, Casas AS, Bisig C, Petri-Fink A (2019) Assessment of the potential for in-plume sulphur dioxide gas-ash interactions to influence the respiratory toxicity of volcanic ash. Environ Res 179:108798. https://doi.org/10.1016/j.envres.2019.108798
Tomašek I, Damby DE, Andronico D, Baxter PJ, Boonen I, Claeys P et al (2021) Assessing the biological reactivity of organic compounds on volcanic ash: implications for human health hazard. Bull Volcanol 83:30. https://doi.org/10.1007/s00445-021-01453-4
Tomašek I, Damby DE, Stewart C, Horwell CJ, Plumlee G, Ottley CJ, Delmelle P, Morman S, El Yazidi S, Claeys P, Kervyn M, Elskens M, Leermakers M (2021b) Development of a simulated lung fluid leaching method to assess the release of potentially toxic elements from volcanic ash. Chemosphere 278:130303. https://doi.org/10.1016/j.chemosphere.2021.130303
Trisnawati I, Budiono E, Sumardi S, Setiadi A (2015) Traumatic inhalation due to Merapi Volcanic Ash. Acta Medica Indonesiana 47:3
Viane C, Bhugwant C, Sieja B, Staudacher T, Demoly P (2009) Comparative study of the volcanic gas emissions and the hospitalizations for asthma of the Reunion Island population between 2005 and 2007. Revue Française d'Allergologie 49:346–351. https://doi.org/10.1016/j.reval.2009.02.010
Webb E, Stewart C, Sami E, Kelsey S, Fairbairn Dunlop P, Dennison E (2021) Variability of naturally occurring fluoride in diverse community drinking water sources, Tanna Island, Vanuatu. Water, Hygiene and Sanitation for Development 11:591–599. https://doi.org/10.2166/washdev.2021.270
Whitty RCW, Ilyinskaya E, Mason E, Wieser PE, Liu EJ, Schmidt A, Roberts T, Pfeffer MA, Brooks B, Mather TA, Edmonds M, Elias T, Schneider DJ, Oppenheimer C, Dybwad A, Nadeau PA, Kern C (2020) Spatial and temporal variations in SO 2 and PM 2.5 levels around Kīlauea Volcano, Hawai’i During 2007–2018. Front Earth Sci 8. https://doi.org/10.3389/feart.2020.00036
Wilson TM, Cole JW, Stewart C, Cronin S, Johnston DM (2011) Ash storms: impacts of wind-remobilised volcanic ash on rural communities and agriculture following the 1991 Hudson eruption, southern Patagonia, Chile. Bulletin of Volcanology 73:223–239. https://doi.org/10.1007/s00445-010-0396-1
Wilson T, Stewart C, Bickerton H, Baxter P, Outes AV, Villarosa G, Rovere E (2013) Impacts of the June 2011 Puyehue-Cordón Caulle volcanic complex eruption on urban infrastructure, agriculture and public health. GNS Science Report 2012/20, p 88. https://www.gns.cri.nz/static/pubs/2012/SR%202012-020%20Print%20Copy.pdf
Witham C, Barsotti S, Dumont S, Oddson B, Sigmundsson F (2020) Practising an explosive eruption in Iceland: outcomes from a European exercise. J Appl Volcanol 9:1. https://doi.org/10.1186/s13617-019-0091-7
World Health Organization (2013) Regional Office for Europe. Review of evidence on health aspects of air pollution: REVIHAAP Project Technical Report, p 302
Wygel CM, Peters SC, McDermott JM, Sahagian DL (2019) Bubbles and dust: experimental results of dissolution rates of metal salts and glasses from volcanic ash deposits in terms of surface area, chemistry, and human health impacts. GeoHealth 3:338–355. https://doi.org/10.1029/2018GH000181
Download references
Acknowledgements
The authors sincerely thank Pierre-Yves Tournigand for graphic design of the manuscript figure. We also thank two anonymous reviewers and John Ewert of the U.S. Geological Survey for their review comments, which have improved this manuscript. Any use of trade, firm or product names is for descriptive purposes only and does not imply endorsement by the U.S. Government.
CS acknowledges funding from New Zealand’s Resilience to Nature’s Challenges National Science Challenge. IT acknowledges the support received from the Agence Nationale de la Recherche of the French government through the program “Investissements d’Avenir” (16-IDEX-0001 CAP 20-25). AS acknowledges funding from Natural Environment Research Council grants NE/S00436X/1 and NE/T006897/1.
Author information
Authors and affiliations.
School of Health Sciences, Massey University, PO Box 756, Wellington, 6021, New Zealand
Carol Stewart
U.S. Geological Survey, Volcano Science Center, Menlo Park, CA, USA
David E. Damby
Institute of Hazard, Risk & Resilience, Department of Earth Sciences, Lower Mountjoy, Durham University, Durham, DH1 3LE, UK
Claire J. Horwell
U.S. Geological Survey, Hawaiian Volcano Observatory, Hilo, HI, USA
Tamar Elias
School of Earth and Environment, University of Leeds, Leeds, UK
Evgenia Ilyinskaya
Laboratoire Magmas et Volcans (LMV), CNRS, IRD, OPGC, Université Clermont Auvergne, Clermont-Ferrand, France
Ines Tomašek
Institute of Genetic Reproduction and Development (iGReD), Translational Approach to Epithelial Injury and Repair Team, CNRS UMR 6293, INSERM U1103, Université Clermont Auvergne, Clermont-Ferrand, France
Orvis School of Nursing, University of Nevada, Reno, NV, USA
Bernadette M. Longo
Centre for Atmospheric Science, Department of Chemistry, University of Cambridge, Cambridge, UK
Anja Schmidt
Department of Geography, University of Cambridge, Cambridge, UK
Department Environment and Natural Resources, University of Iceland, Reykjavík, Iceland
Hanne Krage Carlsen
Department of Occupational and Environmental Medicine, School of Public Health and Community Medicine, Sahlgrenska Academy, Gothenburg University, Gothenburg, Sweden
Department of Earth Sciences, University of Cambridge, Downing Street, Cambridge, Cambridgeshire, CB2 3EQ, UK
Emily Mason
Department of Public Health and Primary Care, University of Cambridge, Downing Street, Cambridge, Cambridgeshire, CB2 3EQ, UK
Peter J. Baxter
School of Environment, University of Auckland, Private Bag 92019, Auckland, 1142, New Zealand
Shane Cronin
Met Office, FitzRoy Road, Exeter, EX1 3PB, UK
Claire Witham
You can also search for this author in PubMed Google Scholar
Contributions
CS formulated the initial proposal. CS and DED coordinated the content. CS, DED, CJH, and TE wrote parts of, and extensively revised, the manuscript. EI, IT, AS, HKC, EM, BML, PJB, and CW wrote part of the manuscript and/or assisted with the preparation of tables and figures (Table 1 IT; Table 2 BML). SC contributed to conceptual development and review. All authors read and approved the final manuscript.
Corresponding author
Correspondence to Carol Stewart .
Ethics declarations
Conflict of interest.
The authors declare no competing interests.
Additional information
Editorial responsibility: K.V. Cashman; Deputy Executive Editor: L. Pioli
This paper constitutes part of a topical collection:
Looking Backwards and Forwards in Volcanology:
A Collection of Perspectives on the Trajectory of a Science
Rights and permissions
Open Access This article is licensed under a Creative Commons Attribution 4.0 International License, which permits use, sharing, adaptation, distribution and reproduction in any medium or format, as long as you give appropriate credit to the original author(s) and the source, provide a link to the Creative Commons licence, and indicate if changes were made. The images or other third party material in this article are included in the article's Creative Commons licence, unless indicated otherwise in a credit line to the material. If material is not included in the article's Creative Commons licence and your intended use is not permitted by statutory regulation or exceeds the permitted use, you will need to obtain permission directly from the copyright holder. To view a copy of this licence, visit http://creativecommons.org/licenses/by/4.0/ .
Reprints and permissions
About this article
Stewart, C., Damby, D.E., Horwell, C.J. et al. Volcanic air pollution and human health: recent advances and future directions. Bull Volcanol 84 , 11 (2022). https://doi.org/10.1007/s00445-021-01513-9
Download citation
Received : 24 July 2021
Accepted : 02 November 2021
Published : 21 December 2021
DOI : https://doi.org/10.1007/s00445-021-01513-9
Share this article
Anyone you share the following link with will be able to read this content:
Sorry, a shareable link is not currently available for this article.
Provided by the Springer Nature SharedIt content-sharing initiative
- Volcanic emissions
- Air pollution
- Health effects
- Health hazard assessment
- Risk management
- Find a journal
- Publish with us
- Track your research
ENCYCLOPEDIC ENTRY
A volcano is an opening in a planet or moon’s crust through which molten rock and gases trapped under the surface erupt, often forming a hill or mountain.
Volcanic eruption
Volcanic eruptions can create colorful and dramatic displays, such as this eruption of this volcano in the Virunga Moutains of the Democratic Republic of the Congo.
Photograph by Chris Johns
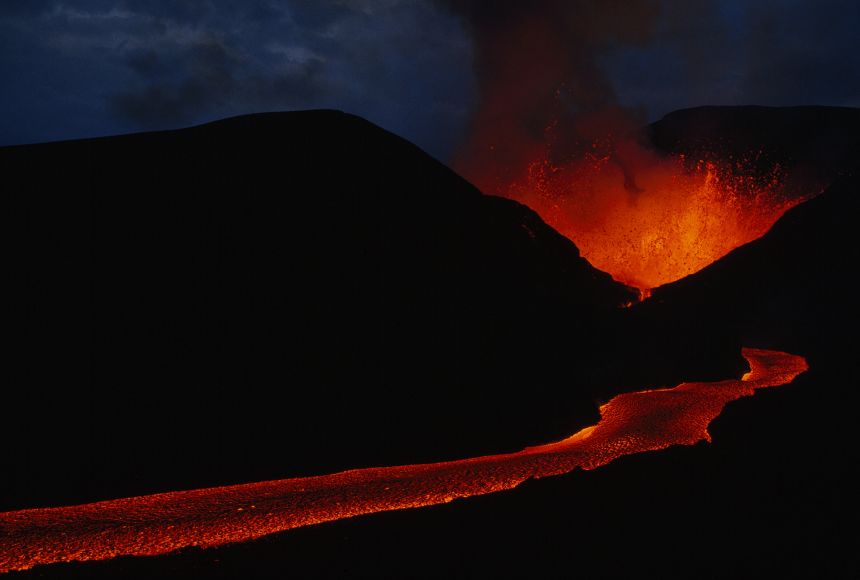
A volcano is an opening in a planet or moon’s crust through which molten rock, hot gases, and other materials erupt . Volcanoes often form a hill or mountain as layers of rock and ash build up from repeated eruptions .
Volcanoes are classified as active, dormant, or extinct. Active volcanoes have a recent history of eruptions ; they are likely to erupt again. Dormant volcanoes have not erupted for a very long time but may erupt at a future time. Extinct volcanoes are not expected to erupt in the future.
Inside an active volcano is a chamber in which molten rock, called magma , collects. Pressure builds up inside the magma chamber, causing the magma to move through channels in the rock and escape onto the planet’s surface. Once it flows onto the surface the magma is known as lava .
Some volcanic eruptions are explosive, while others occur as a slow lava flow. Eruptions can occur through a main opening at the top of the volcano or through vents that form on the sides. The rate and intensity of eruptions, as well as the composition of the magma, determine the shape of the volcano.
Volcanoes are found on both land and the ocean floor. When volcanoes erupt on the ocean floor, they often create underwater mountains and mountain ranges as the released lava cools and hardens. Volcanoes on the ocean floor become islands when the mountains become so large they rise above the surface of the ocean.
Media Credits
The audio, illustrations, photos, and videos are credited beneath the media asset, except for promotional images, which generally link to another page that contains the media credit. The Rights Holder for media is the person or group credited.
Production Managers
Program specialists, last updated.
October 19, 2023
User Permissions
For information on user permissions, please read our Terms of Service. If you have questions about how to cite anything on our website in your project or classroom presentation, please contact your teacher. They will best know the preferred format. When you reach out to them, you will need the page title, URL, and the date you accessed the resource.
If a media asset is downloadable, a download button appears in the corner of the media viewer. If no button appears, you cannot download or save the media.
Text on this page is printable and can be used according to our Terms of Service .
Interactives
Any interactives on this page can only be played while you are visiting our website. You cannot download interactives.
Related Resources
Home — Essay Samples — Environment — Natural Disasters — The Environmental Effects of Volcanoes: A Comprehensive Analysis

The Environmental Effects of Volcanoes: a Comprehensive Analysis
- Categories: Natural Disasters
About this sample

Words: 621 |
Published: Mar 6, 2024
Words: 621 | Page: 1 | 4 min read
Table of contents
Air pollution and climate change, positive effects of volcanic activity, mitigating the environmental impacts of volcanoes.
- The release of gases and particulate matter into the atmosphere
- Global cooling caused by volcanic ash blocking out sunlight
- Contribution to air pollution and respiratory problems
- Impact on climate change through the release of carbon dioxide
- Creation of new landmasses supporting new ecosystems
- Increased biodiversity on newly formed landmasses
- Contribution to the formation of mineral deposits
- Use of volcanic ash and rocks for various purposes
- Close monitoring of volcanic activity to predict eruptions
- Development of effective mitigation strategies
- Reducing reliance on fossil fuels and supporting renewable energy sources
- Protection of vulnerable ecosystems and biodiversity
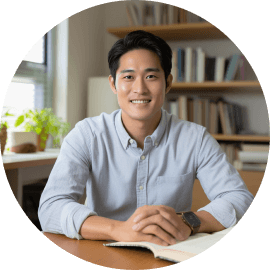
Cite this Essay
Let us write you an essay from scratch
- 450+ experts on 30 subjects ready to help
- Custom essay delivered in as few as 3 hours
Get high-quality help
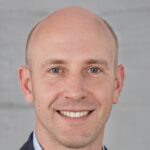
Prof. Kifaru
Verified writer
- Expert in: Environment
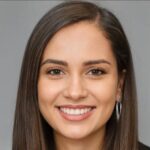
+ 120 experts online
By clicking “Check Writers’ Offers”, you agree to our terms of service and privacy policy . We’ll occasionally send you promo and account related email
No need to pay just yet!
Related Essays
3 pages / 1572 words
2 pages / 892 words
4 pages / 1774 words
1 pages / 566 words
Remember! This is just a sample.
You can get your custom paper by one of our expert writers.
121 writers online
Still can’t find what you need?
Browse our vast selection of original essay samples, each expertly formatted and styled
Related Essays on Natural Disasters
Greek Mythology - Zeus: The Greek God of the Sky, Thunder, and Lightning. (n.d.). Greek Mythology: The Gods, Goddesses, and Heroes Handbook. The Bible: Relevant verses from the books of Romans, Exodus, Revelations, and 2 Samuel. [...]
It was a typical day in my hometown, the sun was shining, and the birds were chirping. I was going about my daily routine when suddenly, the ground beneath my feet started to tremble. At first, I thought it was just a minor [...]
Floods have been a recurring natural disaster that has affected millions of people worldwide. Whether caused by heavy rainfall, snowmelt, or hurricanes, floods have the potential to cause significant damage to property, [...]
Natural disasters have the potential to cause widespread devastation and leave lasting impacts on communities. In recent history, Hurricanes Katrina and Harvey stand out as two of the most destructive and costly hurricanes to [...]
This article is about outdoor fires. For other uses, see Wildfire (disambiguation). For other uses, see Brushfire (disambiguation).The Rim Fire consumed more than 250,000 acres (1,000 km2) of forest near Yosemite National Park, [...]
Have you ever been present in a category 5 hurricane? If the answer is no let me tell you that I just wish you never had to go through an experience like that. In September 20 Hurricane Maria struck Puerto Rico. All the [...]
Related Topics
By clicking “Send”, you agree to our Terms of service and Privacy statement . We will occasionally send you account related emails.
Where do you want us to send this sample?
By clicking “Continue”, you agree to our terms of service and privacy policy.
Be careful. This essay is not unique
This essay was donated by a student and is likely to have been used and submitted before
Download this Sample
Free samples may contain mistakes and not unique parts
Sorry, we could not paraphrase this essay. Our professional writers can rewrite it and get you a unique paper.
Please check your inbox.
We can write you a custom essay that will follow your exact instructions and meet the deadlines. Let's fix your grades together!
Get Your Personalized Essay in 3 Hours or Less!
We use cookies to personalyze your web-site experience. By continuing we’ll assume you board with our cookie policy .
- Instructions Followed To The Letter
- Deadlines Met At Every Stage
- Unique And Plagiarism Free
Thank you for visiting nature.com. You are using a browser version with limited support for CSS. To obtain the best experience, we recommend you use a more up to date browser (or turn off compatibility mode in Internet Explorer). In the meantime, to ensure continued support, we are displaying the site without styles and JavaScript.
- View all journals
- Explore content
- About the journal
- Publish with us
- Sign up for alerts
- 17 August 2022
Huge volcanic eruptions: time to prepare
- Michael Cassidy 0 &
- Lara Mani 1
Michael Cassidy is an associate professor of volcanology at the University of Birmingham, Birmingham, UK.
You can also search for this author in PubMed Google Scholar
Lara Mani is a research associate at the Centre for the Study of Existential Risk, University of Cambridge, UK.
Tonga Geological Services staff making observations of the Hunga Tonga–Hunga Ha‘apai volcano. Credit: Tonga Geological Services/ZUMA/Alamy
The massive eruption of the Hunga Tonga–Hunga Ha‘apai volcano this January in Tonga, in the south Pacific Ocean, was the volcanic equivalent of a ‘near miss’ asteroid whizzing by the Earth. The eruption was the largest since Mount Pinatubo in the Philippines blew in 1991, and the biggest explosion ever recorded by instruments.
Access options
Access Nature and 54 other Nature Portfolio journals
Get Nature+, our best-value online-access subscription
24,99 € / 30 days
cancel any time
Subscribe to this journal
Receive 51 print issues and online access
185,98 € per year
only 3,65 € per issue
Rent or buy this article
Prices vary by article type
Prices may be subject to local taxes which are calculated during checkout
Nature 608 , 469-471 (2022)
doi: https://doi.org/10.1038/d41586-022-02177-x
Mani, L., Tzachor, A. & Cole, P. Nature Commun. 12 , 4756 (2021).
Article PubMed Google Scholar
Lin, J. et al. Clim. Past 18 , 485–506 (2021).
Article Google Scholar
Newhall, C., Self, S. & Robock, A. Geosphere 14 , 572–603 (2018).
Trilling, D. E. et al. Astron. J. 154 , 170 (2017).
Rougier, J., Sparks, R. S. J., Cashman, K. V. & Brown, S. K. Earth Planet. Sci. Lett. 482 , 621–629 (2018).
Aubry, T. J. et al. Nature Commun. 12 , 4708 (2021).
Aubry, T. J. et al. Bull. Volcanol. 84 , 58 (2022).
Mahalingam, A. et al. Impacts of Severe Natural Catastrophes on Financial Markets (Cambridge Centre for Risk Studies, 2018).
Google Scholar
Deligne, N. I., Coles, S. G. & Sparks, R. S. J. J. Geophys. Res. Solid Earth 115 , B06203 (2010).
Giordano, G. & Caricchi, L. Annu. Rev. Earth Planet. Sci. 50 , 231–259 (2022).
Costa, F. et al. Disaster Prev. Manag. 28 , 738–751 (2019).
Pritchard, M. E. et al. J. Appl. Volcanol. 7 , 5 (2018).
Ramsey, M. S., Harris, A. J. L. & Watson, I. M. Bull. Volcanol. 84 , 6 (2021).
Fuglestvedt, J. S., Samset, B. H. & Shine, K. P. Geophys. Res. Lett. 41 , 8627–8635 (2014).
Download references
Reprints and permissions
Supplementary Information
- Further reading
Competing Interests
The authors declare no competing interests.
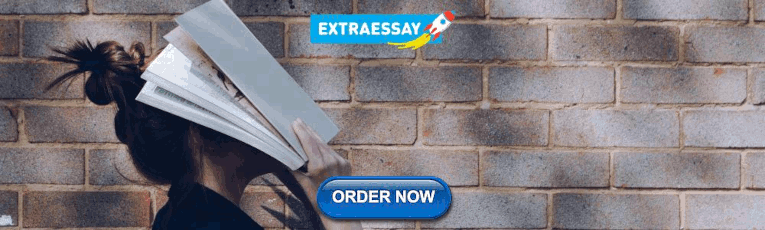
Related Articles
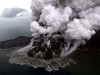
- Volcanology
- Scientific community
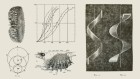
The biologist who built a Faraday cage for a crab
News & Views 09 APR 24
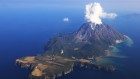
Submerged volcano’s eruption was the biggest since the last ice age
Research Highlight 29 FEB 24
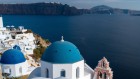
Santorini’s volcanic past: underwater clues reveal giant prehistoric eruption
News Feature 06 FEB 24
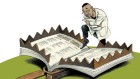
I’m worried I’ve been contacted by a predatory publisher — how do I find out?
Career Feature 15 MAY 24
Standardized metadata for biological samples could unlock the potential of collections
Correspondence 14 MAY 24
Real-world plastic-waste success stories can help to boost global treaty
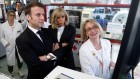
A DARPA-like agency could boost EU innovation — but cannot come at the expense of existing schemes
Editorial 14 MAY 24
Japan can embrace open science — but flexible approaches are key
Correspondence 07 MAY 24
Postdoc Fellowships
Train with world-renowned cancer researchers at NIH? Consider joining the Center for Cancer Research (CCR) at the National Cancer Institute
Bethesda, Maryland
NIH National Cancer Institute (NCI)
Faculty Recruitment, Westlake University School of Medicine
Faculty positions are open at four distinct ranks: Assistant Professor, Associate Professor, Full Professor, and Chair Professor.
Hangzhou, Zhejiang, China
Westlake University
PhD/master's Candidate
PhD/master's Candidate Graduate School of Frontier Science Initiative, Kanazawa University is seeking candidates for PhD and master's students i...
Kanazawa University
Senior Research Assistant in Human Immunology (wet lab)
Senior Research Scientist in Human Immunology, high-dimensional (40+) cytometry, ICS and automated robotic platforms.
Boston, Massachusetts (US)
Boston University Atomic Lab
Postdoctoral Fellow in Systems Immunology (dry lab)
Postdoc in systems immunology with expertise in AI and data-driven approaches for deciphering human immune responses to vaccines and diseases.
Sign up for the Nature Briefing newsletter — what matters in science, free to your inbox daily.
Quick links
- Explore articles by subject
- Guide to authors
- Editorial policies
- Earth Science
Volcano Eruption
Volcanoes are ruptures in the crust of our planet Earth that allow hot gases, molten lava and some rock fragments to erupt by opening and exposing the magma inside. In this piece of article, we will be discussing how and why volcanoes erupt.
How Do Volcanoes Erupt?
It is so hot deep within the earth that some rocks slowly melt and turn into a thick flowing matter known as magma. Since it is lighter than solid rock, the magma rises and collects in magma chambers. Eventually, some magma pushes through fissures and vents on the earth’s surface. Hence, a volcanic eruption occurs, and the erupted magma is known as lava.
We need to understand the Earth’s structure to know how volcanoes erupt. At the top lies the lithosphere, the outermost layer that consists of the upper crust and mantle. The thickness of the crust ranges from 10km to 100km in mountainous locations and mainly consists of silicate rock.
See the video below to know more about the causes of volcanic eruptions.
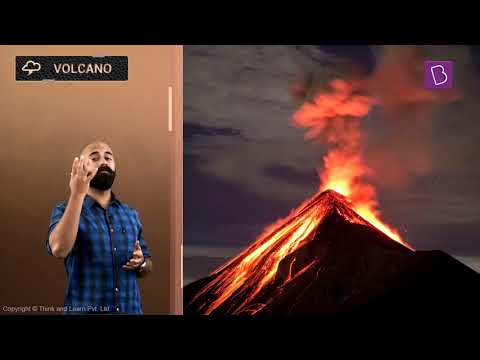
Why Do Volcanoes Erupt?
The Earth’s mantle within the crust is classified into different sections depending on individual seismology. These include the upper mantle, which ranges between 8 – 35 km to 410 km; the transition zone ranges from 400 to 660 km; the lower mantle lies between 660 – 2891 km.
The conditions change dramatically from the crust to the mantle location. The pressures rise drastically and temperatures rise up to 1000 o C. This viscous and molten rock gets collected into large chambers within the Earth’s crust.
Since magma is lighter than surrounding rock, it floats up towards the surface and seeks out cracks and weakness in the mantle. It finally explodes from the peak point of a volcano after reaching the surface. When it is under the surface, the melted rock is known as magma and erupts as ash when comes up.
Rocks, lava and ash are built across the volcanic vent with every eruption. The nature of the eruption mainly depends on the viscosity of the magma. The lava travels far and generates broad shield volcanoes when it flows easily. When it is too thick, it makes a familiar cone volcano shape. If the lava is extremely thick, it can build up in the volcano and explode, known as lava domes.
Causes of Volcanic Eruption
We know that the mantle of the Earth is too hot, and the temperature ranges from 1000° Celsius to 3000° Celsius. The rocks present inside melt due to high pressure and temperature. The melted substance is light in weight. This thin lava comes up to the crust since it can float easily. Since the density of the magma between the area of its creation and the crust is less than the enclosed rocks, the magma gets to the surface and bursts. The magma is composed of andesitic and rhyolitic components along with water, sulfur dioxide, and carbon dioxide in dissolved form. By forming bubbles, excess water is broken up with magma. When the magma comes closer to the surface, the level of water decreases and the gas/magma rises in the channel. When the volume of the bubbles formed is about 75%, the magma breaks into pyroclasts and bursts out. The three main causes of volcanic eruptions are: The buoyancy of the magma Pressure from the exsolved gases in the magma Increase in pressure on the chamber lid Hope you are familiar with why volcanoes erupt and the cause of the volcanic eruption. Stay tuned to BYJU’S to learn about types of volcanoes, igneous rocks, and much more.
Frequently Asked Questions – FAQs
What is lava.
When a volcanic eruption occurs, the erupted magma is known as lava.
State true or false: The nature of the eruption mainly depends on the viscosity of the magma.
What are the causes of volcanic eruption.
The causes of the volcanic eruption are:
- The buoyancy of the magma
- Pressure from the dissolved gases in the magma
- Increase in pressure on the chamber lid
Define magma.
How is earth’s mantle classified.
- The upper mantle – ranges between 8 – 35 km to 410 km
- Transition zone ranges from 400 to 660 km
- Lower mantle lies between 660 – 2891 km
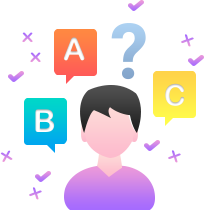
Put your understanding of this concept to test by answering a few MCQs. Click ‘Start Quiz’ to begin!
Select the correct answer and click on the “Finish” button Check your score and answers at the end of the quiz
Visit BYJU’S for all Physics related queries and study materials
Your result is as below
Request OTP on Voice Call
Leave a Comment Cancel reply
Your Mobile number and Email id will not be published. Required fields are marked *
Post My Comment

- Share Share
Register with BYJU'S & Download Free PDFs
Register with byju's & watch live videos.

Volcano World
- Central Andes (Under Construction)
- Hawaiian Volcanism
- Mount Saint Helens
- Volcanoes of Japan
- Extraterrestrial Volcanoes
- Teaching Resources
- Mount St Helens Living Lab
- Plate Tectonics
- Rocks and Minerals
- Prehistoric Earth
- Types of Volcanoes
- Eruption Styles
- Submarine Volcanoes
- Columnar Jointing
- Hydrovolcanism
- Volcanogenic Tsunamis
- Volcanic Minerals
- Volcanic Gases
- Volcanic Lightning
- Volcanic Sounds
- Volcanic Hazards
- GVP Current Eruption Reports
- US Volcano Activity Map
- Volcano Factoids
- Building Volcano Models
- Interviews with Volcanologists
- Working on Volcanoes
- Art Gallery
- Volcano Games
- Mount St. Helens by Helicopter
- Virtual Altiplano
- Virtual Altiplano Bolivia
- Around Santorini
- Climbing Mt Asama
- Driving Kilauea Rim Drive
- Exploring the Canary Islands
- Hiking Mount Ijen
- To the Top of Beerenberg
- Volcano Folklore
- Volcanic Parks
- Volcano Gallery
You are here
How do volcanoes affect people.
Volcanoes affect people in many ways, some are good, some are not. Some of the bad ways are that houses, buildings, roads, and fields can get covered with ash. As long as you can get the ash off (especially if it is wet), your house may not collapse, but often the people leave because of the ash and are not around to continually clean off their roofs. If the ashfall is really heavy it can make it impossible to breathe.
Lava flows are almost always too slow to run over people, but they can certainly run over houses, roads, and any other structures.
Pyroclastic flows are mixtures of hot gas and ash, and they travel very quickly down the slopes of volcanoes. They are so hot and choking that if you are caught in one it will kill you. They are also so fast (100-200 km/hour) that you cannot out-run them. If a volcano that is known for producing pyroclastic flows is looking like it may erupt soon, the best thing is for you to leave before it does.
Some of the good ways that volcanoes affect people include producing spectacular scenery, and producing very rich soils for farming.
Water vapor, the most common gas released by volcanoes, causes few problems. Sulfur dioxide, carbon dioxide and hydrogen are released in smaller amounts. Carbon monoxide, hydrogen sulfide, and hydrogen fluoride are also released but typically less than 1 percent by volume.Gases pose the greatest hazard close to the vent where concentrations are greatest. Away from the vent the gases quickly become diluted by air. For most people even a brief visit to a vent is not a health hazard. However, it can be dangerous for people with respiratory problems.
The continuous eruption at Kilauea presents some new problems. Long term exposure to volcanic fumes may aggravate existing respiratory problems. It may also cause headaches and fatigue in regularly healthy people. The gases also limit visibility, especially on the leeward side of the island where they become trapped by atmospheric conditions.
Source of Information: Volcanic and Seismic Hazards on the Island of Hawaii by Christina Heliker, 1991, U.S. Geological Survey General Interest Publication.
A deadly eruption
The 1815 explosive eruption of Tambora volcano in Indonesia and the subsequent caldera collapse produced 9.5 cubic miles (40 cubic kilometers) of ash. The eruption killed 10,000 people. An additional 80,000 people died from crop loss and famine.
To put it mildly, ash is bad for jet aircraft engines. Apparently the problem is much more severe for modern jet engines which burn hotter than the older ones. Parts of these engines operate at temperatures that are high enough to melt ash that is ingested. Essentially you end up with tiny blobs of lava inside the engine. This is then forced back into other parts where the temperatures are lower and the stuff solidifies. As you can imagine this is pretty bad. One problem that I heard about is that pilots start losing power and apply the throttle, causing the engine to be even hotter and melt more ash.Added to this is the fact that ash is actually tiny particles of glass plus small mineral shards–pretty abrasive stuff. You can imagine that dumping a whole bunch of abrasive powder into a jet engine is not good for the engine. This has been a pretty non-scientific explanation of the problem. I just found an article that describes the problem a little more technically.
“The ash erodes sharp blades in the compressor, reducing its efficiency. The ash melts in the combustion chamber to form molten glass. The ash then solidifies on turbine blades, blocking air flow and causing the engine to stall.” This comes from the FAA Aviation Safety Journal, Vol. 2, No. 3.
Safe distance
The distance you have to evacuate depends entirely on what kind of eruption is going on. For example, Pinatubo, one of the largest recent eruptions sent pyroclastic flows at least 18 km down its flanks, and pumice falls were hot and heavy even beyond that. For example, pumice 7 cm across fell at Clark Air base which is 25 km from the volcano! A 7 cm pumice won’t necessarily kill you but it does mean that there is a lot of pumice falling, and if you don’t get out and continuously sweep off your roof it may fall in and you’ll get squashed.On the other hand, the current eruption at Ruapehu is relatively small. In fact, there were skiers up on the slopes when the eruptions commenced, and even though they were only 1-2 km from the vent they managed to escape. The volcanologists routinely go up on the higher slopes of Ruapehu during these ongoing eruptions to collect ash and take photographs.
So you see, you need to know something about what you think the volcano is going to do before you decide how far to run away. I guess if you have no idea of what the volcano is planning, and have no idea of what it has done in the past, you might want to be at least 25-30 km away, make sure you have a good escape route to get even farther away if necessary, and by all means stay out of low-lying areas!
Cities and Towns

The effect an eruption will have on a nearby city could vary from none at all to catastrophic. For example, atmospheric conditions might carry ash away from the city or topography might direct lahars and pyroclastic flows to unpopulated areas. In contrast, under certain atmospheric, eruption and/or topographic conditions, lahars, pyroclastic flows, and/or ash fall could enter the city causing death and destruction.
This scenario brings up several interesting problems. How do you evacuate a large population if there is little warning before the eruption? Where do these people go? If an eruption is highly likely yet hasn’t happened yet how long can people be kept away from their homes and businesses?
I should point out that in most volcanic crises geologists advise local civil defense authorities. The civil defense authorities decide what to do concerning evacuations, etc.
The IAVCEI has a program to promote research on “Decade” Volcanoes. Decade volcanoes are likely to erupt in the near future and are near large population centers. Mount Rainier in Washington and Mauna Loa in Hawaii are two Decade volcanoes in the U.S. Other Decade volcanoes include Santa Maria, Stromboli, Pinatubo, and Unzen.
What happens to the towns around a volcano when it erupts depends on many things. It depends of the size and type of eruption and the size and location of the town. A few examples might help. The 1984 eruption of Mauna Loa in Hawaii sent lava towards Hilo but the eruption stopped before the flows reached the town. The 1973 eruption of Heimaey in Iceland buried much of the nearby town of Heimaey under lava and cinder. The 1960 eruption of Kilauea in Hawaii buried all of the nearby town of Kapoho under lava and cinder. In 1980, ash from Mount St. Helens fell on many towns in Washington and Oregon. The 1902 eruption of Mount Pelee on the island of Martinique destroyed the town of Saint Pierre with pyroclastic flows. In 1985, the town of Armero was partially buried by lahars generated on Ruiz. For more examples see Decker and Decker (1989).
Mount Mayon , in the Philippines, is a classic example of a stratovolcano. Image credit: Steve O’Meara
Source of Information: Decker, R., and Decker, B., 1989, Volcanoes: W.H. Freeman, New York, 285 p.
Contact Info
VW is a higher education, k-12, and public outreach project of the Oregon Space Grant Consortium administered through the Department of Geosciences at Oregon State University.
Disclaimers and Policies | Login
- Growth & Development
- Play & Activities
- Life Skills
- Play & Learning
- Learning & Education
- Rhymes & Songs
- Preschool Locator

Essay On The Volcano – 10 Lines, Short & Long Essay For Kids
Key Points To Remember When Writing An Essay On The Volcano For Lower Primary Classes
10 lines on the volcano for kids, a paragraph on the volcano for children, short essay on volcano in 200 words for kids, long essay on volcano for children, interesting facts about volcanoes for children, what will your child learn from this essay.
A volcano is a mountain formed through an opening on the Earth’s surface and pushes out lava and rock fragments through that. It is a conical mass that grows large and is found in different sizes. Volcanoes in Hawaiian islands are more than 4000 meters above sea level, and sometimes the total height of a volcano may exceed 9000 meters, depending on the region it is found. Here you will know and learn how to write an essay on a volcano for classes 1, 2 & 3 kids. We will cover writing tips for your essay on a volcano in English and some fun facts about volcanoes in general.
Volcanoes are formed as a result of natural phenomena on the Earth’s surface. There are several types of volcanoes, and each may emit multiple gases. Below are some key points to remember when writing an essay on a volcano:
- Start with an introduction about how volcanoes are formed. How they impact the Earth, what they produce, and things to watch out for.
- Discuss the different types of volcanoes and talk about the differences between them.
- Cover the consequences when volcanoes erupt and the extent of the damage on Earth.
- Write a conclusion paragraph for your essay and summarise it.
When writing a few lines on a volcano, it’s crucial to state interesting facts that children will remember. Below are 10 lines on volcanoes for an essay for classes 1 & 2 kids.
- Some volcanoes erupt in explosions, and then some release magma quietly.
- Lava is hot and molten red in colour and cools down to become black in colour.
- Hot gases trapped inside the Earth are released when a volcano erupts.
- A circle of volcanoes is referred to as the ‘Ring of Fire.’
- Volcano formations are known as seismic activities.
- Active volcanoes are spread all across the earth.
- Volcanoes can remain inactive for thousands of years and suddenly erupt.
- Most volcanic eruptions occur underwater and result from plates diverging from the margins.
- Volcanic hazards happen in the form of ashes, lava flows, ballistics, etc.
- Volcanic regions have turned into tourist attractions such as the ones in Hawaii.
Volcanoes can be spotted at the meeting points of tectonic plates. Like this, there are tons of interesting facts your kids can learn about volcanoes. Here is a short paragraph on a volcano for children:
A volcano can be defined as an opening in a planet through which lava, gases, and molten rock come out. Earthquake activity around a volcano can give plenty of insight into when it will erupt. The liquid inside a volcano is called magma (lava), which can harden. The Roman word for the volcano is ‘vulcan,’ which means God of Fire. Earth is not the only planet in the solar system with volcanoes; there is one on Mars called the Olympus Mons. There are mainly three types of volcanoes: active, dormant, and extinct. Some eruptions are explosive, and some happen as slow-flowing lava.
Small changes occur in volcanoes, determining if the magma is rising or not flowing enough. One of the common ways to forecast eruptions is by analysing the summit and slopes of these formations. Below is a short essay for classes 1, 2, & 3:
As a student, I have always been curious about volcanoes, and I recently studied a lot about them. Do you know? Krakatoa is a volcano that made an enormous sound when it exploded. Maleo birds seek refuge in the soil found near volcanoes, and they also bury their eggs in these lands as it keeps the eggs warm. Lava salt is a popular condiment used for cooking and extracted from volcanic rocks. And it is famous for its health benefits and is considered superior to other forms of rock or sea salts. Changes in natural gas composition in volcanoes can predict how explosive an eruption can be. A volcano is labelled active if it constantly generates seismic activity and releases magma, and it is considered dormant if it has not exploded for a long time. Gas bubbles can form inside volcanoes and blow up to 1000 times their original size!
Volcanic eruptions can happen through small cracks on the Earth’s surface, fissures, and new landforms. Poisonous gases and debris get mixed with the lava released during these explosions. Here is a long essay for class 3 kids on volcanoes:
Lava can come in different forms, and this is what makes volcanoes unique. Volcanic eruptions can be dangerous and may lead to loss of life, damaging the environment. Lava ejected from a volcano can be fluid, viscous, and may take up different shapes.
When pressure builds up below the Earth’s crust due to natural gases accumulating, that’s when a volcanic explosion happens. Lava and rocks are shot out from the surface to make room on the seafloor. Volcanic eruptions can lead to landslides, ash formations, and lava flows, called natural disasters. Active volcanoes frequently erupt, while the dormant ones are unpredictable. Thousands of years can pass until dormant volcanoes erupt, making their eruption unpredictable. Extinct volcanoes are those that have never erupted in history.
The Earth is not the only planet in the solar system with volcanoes. Many volcanoes exist on several other planets, such as Mars, Venus, etc. Venus is the one planet with the most volcanoes in our solar system. Extremely high temperatures and pressure cause rocks in the volcano to melt and become liquid. This is referred to as magma, and when magma reaches the Earth’s surface, it gets called lava. On Earth, seafloors and common mountains were born from volcanic eruptions in the past.
What Is A Volcano And How Is It Formed?
A volcano is an opening on the Earth’s crust from where molten lava, rocks, and natural gases come out. It is formed when tectonic plates shift or when the ocean plate sinks. Volcano shapes are formed when molten rock, ash, and lava are released from the Earth’s surface and solidify.
Types Of Volcanoes
Given below various types of volcanoes –
1. Shield Volcano
It has gentle sliding slopes and ejects basaltic lava. These are created by the low-viscosity lava eruption that can reach a great distance from a vent.
2. Composite Volcano (Strato)
A composite volcano can stand thousands of meters tall and feature mudflow and pyroclastic deposits.
3. Caldera Volcano
When a volcano explodes and collapses, a large depression is formed, which is called the Caldera.
4. Cinder Cone Volcano
It’s a steep conical hill formed from hardened lava, tephra, and ash deposits.
Causes Of Volcano Eruptions
Following are the most common causes of volcano eruptions:
1. Shifting Of Tectonic Plates
When tectonic plates slide below one another, water is trapped, and pressure builds up by squeezing the plates. This produces enough heat, and gases rise in the chambers, leading to an explosion from underwater to the surface.
2. Environmental Conditions
Sometimes drastic changes in natural environments can lead to volcanoes becoming active again.
3. Natural Phenomena
We all understand that the Earth’s mantle is very hot. So, the rock present in it melts due to high temperature. This thin lava travels to the crust as it can float easily. As the area’s density is compromised, the magma gets to the surface and explodes.
How Does Volcano Affect Human Life?
Active volcanoes threaten human life since they often erupt and affect the environment. It forces people to migrate far away as the amount of heat and poisonous gases it emits cannot be tolerated by humans.
Here are some interesting facts:
- The lava is extremely hot!
- The liquid inside a volcano is known as magma. The liquid outside is called it is lava.
- The largest volcano in the solar system is found on Mars.
- Mauna Loa in Hawaii is the largest volcano on Earth.
- Volcanoes are found where tectonic plates meet and move.
Your child will learn a lot about how Earth works and why volcanoes are classified as natural disasters, what are their types and how they are formed.
Now that you know enough about volcanoes, you can start writing the essay. For more information on volcanoes, be sure to read and explore more.
Tsunami Essay for Kids Essay on Earthquake in English for Children How to Write An Essay On Environmental Pollution for Kids
- Essays for Class 1
- Essays for Class 2
- Essays for Class 3
5 Recommended Books To Add To Your Child’s Reading List and Why
5 absolute must-watch movies and shows for kids, 15 indoor toys that have multiple uses and benefits, leave a reply cancel reply.
Log in to leave a comment

Most Popular
The best toys for newborns according to developmental paediatricians, the best toys for three-month-old baby brain development, recent comments.

FirstCry Intelli Education is an Early Learning brand, with products and services designed by educators with decades of experience, to equip children with skills that will help them succeed in the world of tomorrow.
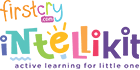
The FirstCry Intellikit `Learn With Stories` kits for ages 2-6 brings home classic children`s stories, as well as fun activities, specially created by our Early Learning Educators.
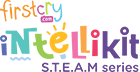
For children 6 years and up, explore a world of STEAM learning, while engaging in project-based play to keep growing minds busy!
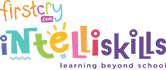
Build a love for reading through engaging book sets and get the latest in brain-boosting toys, recommended by the educators at FirstCry Intellitots.
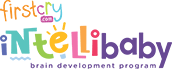
Our Comprehensive 2-year Baby Brain Development Program brings to you doctor-approved toys for your baby`s developing brain.
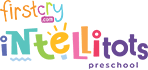
Our Preschool Chain offers the best in education across India, for children ages 2 and up.
©2024 All rights reserved
- Privacy Policy
- Terms of Use
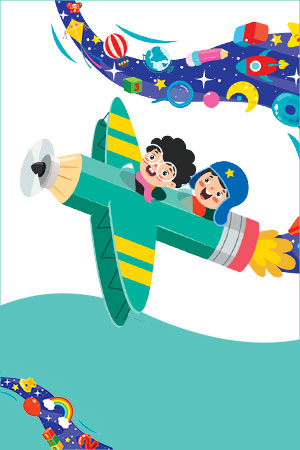
Welcome to the world of Intelli!
We have some FREE Activity E-books waiting for you. Fill in your details below so we can send you tailor- made activities for you and your little one.
Parent/Guardian's Name
Child's DOB
What would you like to receive other than your Free E-book? I would like information, discounts and offers on toys, books and products I want to find a FirstCry Intellitots Preschool near me I want access to resources for my child's development and/or education
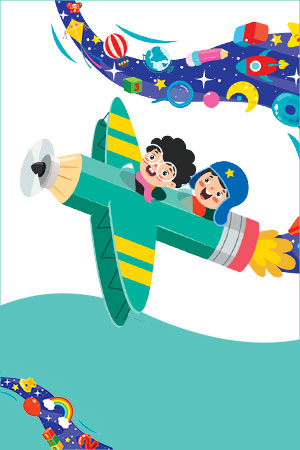
Welcome to the world of intelli!
FREE guides and worksheets coming your way on whatsapp. Subscribe Below !!

THANK YOU!!!
Here are your free guides and worksheets.
Volcanic Eruptions: Understanding the Causes and Impact on Earth's Surface
Volcanic eruptions are among the most captivating and awe-inspiring natural events on Earth. These explosive releases of magma and gases from the depths of the planet have shaped landscapes, influenced climates, and left a lasting impact on both the environment and human civilization. This essay delves into the causes of volcanic eruptions, their mechanisms, and their far-reaching consequences on Earth's surface and beyond.
Volcanic eruptions are primarily driven by the movement of molten rock, known as magma, from the Earth's mantle towards the surface. Magma is generated through the partial melting of the Earth's mantle in regions called magma chambers. As the magma accumulates, pressure builds up within the chamber until it reaches a critical point, leading to an explosive release of gases and molten material.
The type of volcanic eruption is influenced by the composition of the magma and the presence of gases within it. Magma with high silica content tends to be more viscous, leading to explosive eruptions characterized by pyroclastic flows and ash plumes. On the other hand, magma with lower silica content is more fluid and tends to result in effusive eruptions, producing lava flows that can extend over great distances.
Volcanic eruptions can occur along divergent boundaries, where tectonic plates move apart and new oceanic crust is formed, as well as along convergent boundaries, where plates collide and subduction occurs. Subduction zones, where one tectonic plate is forced beneath another, are particularly prone to explosive volcanic activity due to the release of water and other volatiles from the subducting plate, which lowers the melting temperature of the overlying mantle.
The impact of volcanic eruptions on Earth's surface and environment can be profound and far-reaching. In the short term, explosive eruptions can lead to devastating pyroclastic flows, which are rapidly moving clouds of hot gas and volcanic debris that can engulf and destroy everything in their path. Ash plumes from volcanic eruptions can reach high into the atmosphere, affecting weather patterns and causing temporary cooling of the planet.
In the long term, volcanic eruptions can shape landscapes and create new landforms. Lava flows from effusive eruptions can create vast lava plateaus and volcanic islands, while explosive eruptions can lead to the formation of volcanic calderas and volcanic mountains. Over time, volcanic ash and other ejected material can enrich the soil, making volcanic regions fertile for agriculture.
The impact of volcanic eruptions on human civilization can be both destructive and beneficial. Throughout history, volcanic eruptions have caused loss of life and property, as well as disruptions to agriculture and trade. The eruption of Mount Tambora in 1815, for example, led to the "Year Without a Summer" in 1816, causing widespread crop failures and food shortages around the world.
On the other hand, volcanic eruptions have also played a role in cultural and religious beliefs, with many ancient societies viewing volcanoes as manifestations of powerful gods or spirits. Volcanic ash has been used in pottery and construction, and volcanic landscapes have been revered as sacred sites in many cultures.
In conclusion, volcanic eruptions are awe-inspiring natural events that have a significant impact on Earth's surface and environment. The movement of magma from the Earth's mantle to the surface drives these explosive releases of gases and molten material. The consequences of volcanic eruptions can be both destructive and beneficial, shaping landscapes, influencing climates, and leaving a lasting impact on human civilization and cultural beliefs. The study of volcanic eruptions continues to be an important area of research, enhancing our understanding of Earth's dynamic and ever-changing geology.
Get started for free

Please enter a valid Name
Please enter a valid email address
Please enter a valid Phone Number
Please enter a valid Password
By creating your account, you agree to the Terms of Service and Privacy Policy .
Already have an account? Sign In here.
Please enter your email address
Show Password
Forgot Password?
Don’t have an account? Sign Up
Verify Your Account
Enter the verification codes to confirm your identity.
Hi, Continue Logging In
Continue Logging In
Verify Your Email
Please check your inbox for a verification code to confirm your identity.
Code sent to [email protected]
Send again in seconds
Code sent to +1 302 385 6690
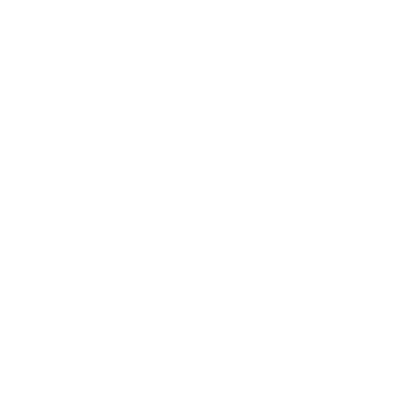
Sample details
Volcanic Eruptions
- Cause and Effect
- Views: 1,744
Related Topics
- Temperature
- Mississippi
- Global Warming
- Precipitation
- Latin America
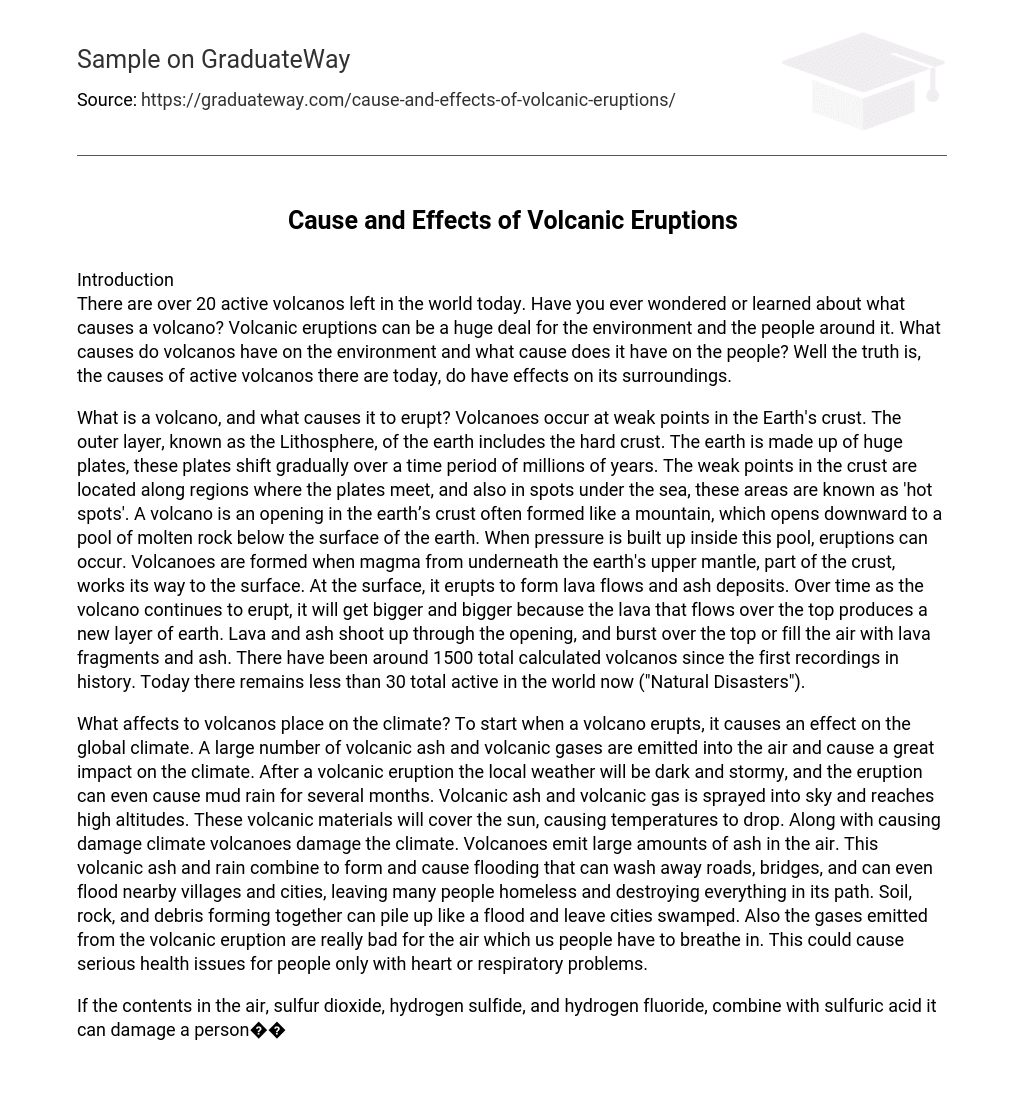
Cause and Effects of Volcanic Eruptions
Introduction There are over 20 active volcanos left in the world today. Have you ever wondered or learned about what causes a volcano? Volcanic eruptions can be a huge deal for the environment and the people around it. What causes do volcanos have on the environment and what cause does it have on the people? Well the truth is, the causes of active volcanos there are today, do have effects on its surroundings.
What is a volcano, and what causes it to erupt? Volcanoes occur at weak points in the Earth’s crust. The outer layer, known as the Lithosphere, of the earth includes the hard crust. The earth is made up of huge plates, these plates shift gradually over a time period of millions of years. The weak points in the crust are located along regions where the plates meet, and also in spots under the sea, these areas are known as ‘hot spots’. A volcano is an opening in the earth’s crust often formed like a mountain, which opens downward to a pool of molten rock below the surface of the earth. When pressure is built up inside this pool, eruptions can occur. Volcanoes are formed when magma from underneath the earth’s upper mantle, part of the crust, works its way to the surface. At the surface, it erupts to form lava flows and ash deposits. Over time as the volcano continues to erupt, it will get bigger and bigger because the lava that flows over the top produces a new layer of earth. Lava and ash shoot up through the opening, and burst over the top or fill the air with lava fragments and ash. There have been around 1500 total calculated volcanos since the first recordings in history. Today there remains less than 30 total active in the world now (“Natural Disasters”).
ready to help you now
Without paying upfront
What affects to volcanos place on the climate? To start when a volcano erupts, it causes an effect on the global climate. A large number of volcanic ash and volcanic gases are emitted into the air and cause a great impact on the climate. After a volcanic eruption the local weather will be dark and stormy, and the eruption can even cause mud rain for several months. Volcanic ash and volcanic gas is sprayed into sky and reaches high altitudes. These volcanic materials will cover the sun, causing temperatures to drop. Along with causing damage climate volcanoes damage the climate. Volcanoes emit large amounts of ash in the air. This volcanic ash and rain combine to form and cause flooding that can wash away roads, bridges, and can even flood nearby villages and cities, leaving many people homeless and destroying everything in its path. Soil, rock, and debris forming together can pile up like a flood and leave cities swamped. Also the gases emitted from the volcanic eruption are really bad for the air which us people have to breathe in. This could cause serious health issues for people only with heart or respiratory problems.
If the contents in the air, sulfur dioxide, hydrogen sulfide, and hydrogen fluoride, combine with sulfuric acid it can damage a person’s skin. (“Climate Effects of Volcanic Eruptions”). Volcanic eruptions have a major impact of the natural landscape of the earth. Land is the world’s most valuable resource. Land is wear millions and millions of plant species grow. Volcanic ash can have a good effect on the environment. Volcanic ash is filled with nutrients that make the soil more fertile which helpful for any potential farmers located around the volcano. However, were ever the lava hardens and turns into rock on the earth will make for useless ground for farmers and anyone else looking to use the land. The rock will take thousands of years to turn back into dirt and become useful. Also, even though it’s a small percentage, the ash from the volcanic eruption makes up 1% of earth’s surface. Volcanoes affect people in many ways, some are good, and others are not. 200,000 people have been reported dead due to a volcanic eruption in the past 500 years. The worst recorded volcanic disaster was believed to cause over 92,000 casualties from falling ash and other related causes. 100,000 more deaths were believed to be caused from starvation in the area. Other ways that volcanos effect people in bad ways are that their houses, buildings, roads, and fields can get covered with ash. Houses and buildings may not always collapse, but often the people affected by the volcano leave because of the ash, and are not there to get the ash off of their roofs. When the raining ash is really heavy, or wet, it can become impossible for people to breathe. People are not going to chased down by flowing lava, but the lava will possibly destroy and certainly run over houses, roads, and any other structures. The only real good affect a volcano has for a human is if you are a farmer. Volcanic ash landing a farmer’s field will produce very rich soils. Volcanos also attract many tourists, which in return brings a lot of jobs to the area. Also once it erupts; if you search within the volcano you can find metallic minerals such as zinc, lead, and even gold. A volcanic eruption will also make for a great scene. It’s not every day a person can watch hot fiery lava bursting into air making for magnificent photos (“How Do Volcanoes Affect People?”). What causes volcanos produces great effects towards many different things. Humans, weather, land, buildings, and even the atmosphere are affected by a volcanic eruption. Volcanos can be good but also bad for humans and the environment. And so it is true, the causes of active volcanos there are today, do have effects on its surroundings.
“Climate Effects of Volcanic Eruptions.” N.p., n.d. Web. 6 Dec. 2012. “Natural Disasters.” ThinkQuest. Oracle Foundation, n.d. Web. 06 Dec. 2012. Decker, R. & B. “How Do Volcanoes Affect People?” Volcano World. Oregon State, 1989. Web. 6 Dec. 2012.
Cite this page
https://graduateway.com/cause-and-effects-of-volcanic-eruptions/
You can get a custom paper by one of our expert writers
- Natural Disaster
- Natural Resources
Check more samples on your topics
The positive and negative effects of volcanic eruptions.
A volcano is an opening in the earth’s crust were magma from deep inside the earth forces its way to the surface. Once the volcano erupts the magma is called lava. Volcanic eruptions have positive and negative effects. For example the lava flow can cause complete chaocs and dismantle villages, but once the lava decompses
Differences Between Basaltic, Rhyolitic and Andesitic Volcanic Eruptions
The goal is to explain the differences between Basaltic, Rhyolitic, and Andesitic volcanic eruptions. This will be done by discussing the processes that cause them, the types of volcanoes they create, and the substances they release.When magma, a scorching liquid rock within the earth's core, rises to the surface, volcanic eruptions occur. This process creates
Differences in Volcanic Hazards
The purpose of this essay is to evaluate the different factors that contribute to the variations in worldwide volcano risks. This analysis will be graded out of 40 marks. A hazard is a situation that poses a certain level of risk to life, health, property, or the environment. The severity of the hazard
Yellowstone Volcanic Activity Sample
Yellowstone National Park is what Geologists call a caldera ; a big crater shaped caused from a volcanic eruption. Unlike volcanic activity that happens due to plate boundaries. Yellowstone is located over a hot spot in North America. It is located in Wyoming. Montana. and Idaho. If you look at a map of the history
Essay – Kilauea Volcanic Crater
The state of Hawaii is composed of multiple islands that form an archipelago. Within this group of islands lies central Hawaii, which includes the highly active volcanic crater named Kilauea. Kilauea is widely recognized for its frequent volcanic activity and is regarded as one of the most active craters globally (Comptons). Craters like Kilauea are
History of the Eruptions of Mount Adams
Mount Adams is a massive volcano in the Cascade Range that surpasses the size of nearby mountains. While it has been less active than St. Helens, Rainier, and Mt. in recent times, Mount Adams remains an important landmark in the area. Hood, it will erupt again. In the future, eruptions will likely occur more frequently from
Cause and Effects of Divorce
When two individuals choose to enter into the sacred bond of marriage, they do so with the hopeful expectation of a lifelong commitment. Unfortunately, marriages oftentimes end in divorce due to various reasons, causing immense stress and turmoil for both partners involved as well as their children. Shockingly, almost 1 million children under the age
Cause & Effects of Bullying
According to many students, schools often fail to effectively address bullying as adults rarely intervene. Bullying negatively impacts victims, bullies, and bystanders by harming their self-esteem, health, and academic success. Those who experience bullying frequently also endure depression which worsens their self-esteem problems and leads to a loss of trust in themselves and others.Various circumstances
Euthanasia (Cause and Effects)
Medical ethics
Euthanasia or mercy killing as we all know it has its own cause and effect. What are the cause of this kind of problem? Well as we all know that pain is the most common reason. Pain because of relationships, pain because of financial problems. Of course suicidal thoughts is one of the reasons for

Hi, my name is Amy 👋
In case you can't find a relevant example, our professional writers are ready to help you write a unique paper. Just talk to our smart assistant Amy and she'll connect you with the best match.
Iceland volcano: What happens if it erupts?
Residents of Grindavik have evacuated the town as the threat of a volcanic eruption looms over Iceland.

Iceland, which has 33 active volcanic systems, declared a state of emergency and ordered the mandatory evacuation of the town of Grindavik on Saturday.
Here is what to know:
Keep reading
Volcanic eruption could destroy icelandic fishing town, say experts, where is grindavik and what is happening there.
Grindavik is a fishing town on Iceland’s southwestern peninsula of Reykjanes. It is home to 3,800 people. Around 900 earthquakes hit southern Iceland on Monday alone, adding to the tens of thousands of tremors that have shaken up the south of the country in recent weeks.
Grindavik residents described being evacuated from their homes by police in the early hours of Saturday as the ground shook, roads cracked and buildings suffered structural damage.
Scientists have linked these earthquakes to the movement and spreading of magma, which is around 5km (3 miles) underground. Land in the region has risen by 9cm since October 27, according to the Icelandic Meteorological Office.
Authorities fear that molten rock can rise to the surface and hit Grindavik. Experts expect an eruption soon since the magma is at a shallow depth.
The Icelandic Meteorological Office said on Monday there was a “significant likelihood” of an eruption in the coming days on or just off Reykjanes, despite the size and intensity of earthquakes decreasing.
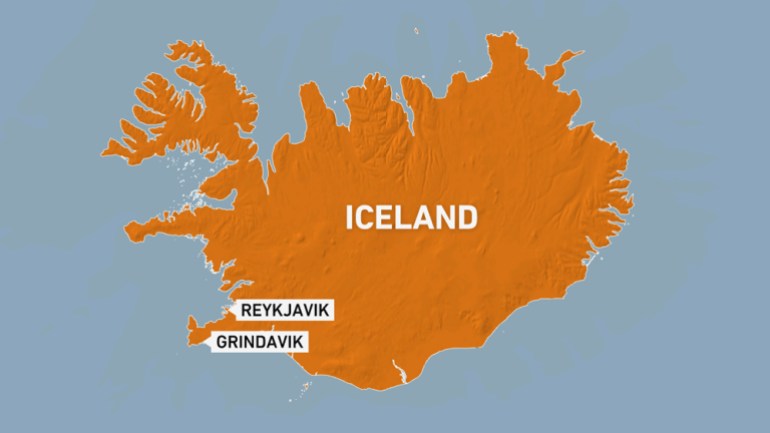
What precautionary measures are being taken?
The residents were told to leave Grindavik on Saturday and were allowed to briefly return on Sunday to collect their belongings including documents, medicines or pets, with Icelandic police and civil protection vehicles on standby.
Almost all of the town’s residents found accommodation with family members or friends. Only between 50 and 70 people were staying at evacuation centres, a rescue official told the Reuters news agency.
The Blue Lagoon geothermal spa, a tourist attraction in Iceland also announced its temporary closure until November 16. The spa is located on the peninsula, “amid moss-covered lava fields”, according to its website.
Scientists are monitoring the situation to examine the flow and proximity of the magma to try to predict the likelihood of eruption.
On Tuesday, the authorities were preparing to build defence walls around a geothermal power plant in the southwestern part of the country that they hope will protect it from lava flows in the event of an eruption.
What can happen if the volcano erupts in Iceland?
The lava can potentially threaten the town of Grindavik and the Blue Lagoon. Geothermal pipelines that supply hot water to thousands of homes are also under threat.
There also might be a risk of toxic fumes and air pollution as a result of the eruption.
“We have a fissure that’s about 15km [9.3 miles] long, and anywhere on that fissure we can see that an eruption could happen,” Vidir Reynisson, head of Iceland’s Civil Protection and Emergency Management told the AFP news agency.
However, the end of the fissure goes into the sea, which means the eruption can occur on the ocean floor, which would likely cause a large ash cloud.
Volcanic eruptions also pose a serious hazard to aviation because they can spew highly abrasive ash high into the atmosphere where it can cause jet engines to fail, damage flight control systems and reduce visibility.
Has this happened in Iceland before?
Iceland sits above a volcanic hotspot in the North Atlantic and averages an eruption every four to five years.
The 2010 eruption of the Eyjafjallajokull volcano was one of the most disruptive eruptions recently, resulting in widespread airspace closures over Europe and costing airlines an estimated $3bn as they cancelled more than 100,000 flights.
After 800 years, three eruptions have taken place on the peninsula of Reykjanes near the Fagradalsfjall volcano: in March 2021, August 2022 and July 2023 . Previous eruptions did not cause damage, having occurred in remote valleys.
Volcanologists believe the new cycle of increased activity could last for several decades or centuries.
Is the capital Reykjavik at risk?
Grindavik is around 40km (25 miles) southwest of Iceland’s capital, Reykjavik, where authorities have not ordered residents to evacuate, indicating that they do not think the country’s biggest city will be impacted by an eruption.
- Share full article
Advertisement
Subscriber-only Newsletter
Climate Forward
The doom vs. optimism debate.
How to reconcile two new reports that seem to tell very different stories about the state of climate change.

By David Gelles
Depending on how you look at it, the climate story is one that can inspire genuine optimism or a fair bit of doomerism.
On any given day, there are news stories , academic studies and memes that support two dueling narratives:
The fatalistic view that global warming is out of control.
And the more hopeful, technocratic outlook that, while the challenges are real, the solutions are within reach.
This week brought yet another slew of data that provides an opportunity to hold these two seemingly contradictory truths at the same time.
There are elements of truth to both perspectives, of course. It’s a disorienting dynamic that we grapple with as reporters covering climate change. Our work involves reporting on the world as it is. Sometimes that means staring down hard facts about the mess we’ve made, and sometimes that means highlighting the real progress that is underway.
Yes, planet-warming emissions are still on the rise, and the effects of climate change are growing worse. And also, yes, renewable energy is coming online at record rates, and there is a huge global effort to deal with an overheating planet.
On Wednesday, a new report highlighted the stunning, record-breaking gains in wind and solar power around the world. A full 30 percent of global electricity was generated by renewables last year, according to Ember , an energy think tank.
On the very same day, however, the latest figures from the National Oceanic and Atmospheric Administration’s Mauna Loa Observatory revealed record levels of carbon dioxide in the atmosphere . This was the largest 12-month increase ever recorded on the so-called Keeling Curve, which has been used to measure the concentration of CO2 since 1958.
To help make sense of this split-screen moment, I turned to Tony Leiserowitz, director of the Yale Program on Climate Change Communication. Leiserowitz, who tracks public perceptions of global warming, is finely attuned to the often jarring work of reconciling conflicting story lines.
“More than one thing can be true at the same time,” he wrote in an email. “We are witnessing astonishing growth in clean energy. Meanwhile, the hourglass of time we have left to limit global warming at ‘safe’ levels is running out.”
‘Take heart in this news’
Gains in clean energy generation is a theme we’ve been tracking for years now.
Solar power has gotten exponentially cheaper over the past decade, thanks largely to low-cost Chinese manufacturing. And in recent years, it has become more affordable to install wind turbines.
The result, according to Ember, was a 23 percent jump in solar power generation last year, and a 10 percent jump in wind generation. Much of the growth came in China. The country accounted for 51 percent of new solar generation and 60 percent of new wind generation.
Global fossil fuel production grew less than 1 percent last year.
“We should take heart in this news,” said Stacey Abrams, the former Georgia state representative who is now senior counsel at Rewiring America, a nonprofit group working to promote clean power. “We now have more evidence that our shift to clean energy sources is the only viable path to healthier, more resilient communities and a safer climate, here and around the globe.”
And it’s not just power generation that is changing for the better. As my colleagues Brad Plumer and Nadja Popovich report, giant batteries are beginning to play a meaningful role in keeping the lights on and stabilizing the grid .
But Abrams cautioned that good news about renewables shouldn’t lead to complacency. “We can’t for a moment slow down,” she said. “These twin reports demonstrate the urgency of action to ratchet down reliance on fossil fuels while speeding delivery of investment in clean generation.”
‘We sadly continue to break records’
The Keeling Curve data offers another reminder, as if one were needed, that planet-warming emissions, driven largely by the burning of fossil fuels, are continuing to transform our planet.
For more than 65 years now, scientific instruments atop volcanoes in Hawaii have monitored how much carbon dioxide is present in the atmosphere. The data “reveals a saw-toothed line that ticks continually upward over time,” my colleague Elena Shao wrote in 2022 . “That pattern is considered by many scientists to be the most important evidence that the climate is changing because of human activity.”
This week’s update was a doozy.
Not only did the concentration of CO2 reach an all-time high, but the trend is accelerating: This was the biggest year-over-year jump ever seen. The NOAA report also said CO2 concentration tends to rise quickly toward the end of El Niño cycles like the one we’re currently experiencing.
Which leads us back to the Ember study. The world is making steady progress in ramping up renewables. And that’s a real cause for optimism.
“The notion of a ‘doomer camp’ is overstated,” Leiserowitz said. “The larger majority of Americans reject a fatalistic view of climate change. In fact, the far larger and more important problem is that most Americans are not worried enough.”
5 more things to know
Trump told oil executives they should give him $1 billion in campaign donations because he planned to roll back regulations. Lisa Friedman, Coral Davenport, Jonathan Swan and Maggie Haberman report today about at a meeting between Trump and oil executives and lobbyists at Mar-a-Lago last month that included leaders from Exxon Mobil, EQT Corporation and the American Petroleum Institute. Lawyers and lobbyists working for the U.S. oil industry are also drawing up ready-to-sign executive orders for Trump, Politico reported . 77 percent of climate scientists believe global temperatures will reach at least 2.5 degrees Celsius, or 4.5 Fahrenheit, above preindustrial levels. That’s from a survey of 380 top climate scientists by The Guardian .
Human-driven changes to the planet are making infectious diseases more dangerous to people, animals and plants, according to a new study . Biodiversity loss is the biggest cause, as well as climate change and invasive species.
Torrential rains have caused one of Brazil’s worst floods in modern history, leaving more than 100 dead in the Rio Grande do Sul State. President Luiz Inácio Lula da Silva announced a $10 billion plan to help the state, which is home to 11 million people and has some towns almost entirely submerged. The floods could cause a spike in food prices, CNN reported , because the state is a major producer of soy, corn and rice.
25 Republican state attorneys general are suing the Environmental Protection Agency to stop the Biden administration’s new coal power plant rules, which require coal-burning plants to limit greenhouse gas emissions. The lawsuit, led by coal-rich West Virginia, argues that the rules contradict a 2022 Supreme Court ruling that curbed the Environmental Protection’s power to cut emissions.
More climate news:
The U.S. Department of Agriculture approved a “climate friendly” label for a Tyson beef brand, but hasn’t shared the data behind the claim despite requests from a watchdog group, Inside Climate News reports .
The Guardian asked scientists about the most powerful climate actions people can take. The answers: Vote for politicians who support strong climate policy, fly less and eat less meat .
The world’s largest direct air capture plant is now live and has started sucking carbon dioxide out of the atmosphere in Iceland, Canary Media reports . Read more about the project in David Gelles’ article from March .
Correction: The April 9 newsletter misstated the source of nitrous oxide, a potent greenhouse gas. Nitrous oxide is produced mainly from natural and agricultural ecosystems, not from aviation.
Thanks for being a subscriber.
Read past editions of the newsletter here .
If you’re enjoying what you’re reading, please consider recommending it to others. They can sign up here . Browse all of our subscriber-only newsletters here . And follow The New York Times on Instagram , Threads , Facebook and TikTok at @nytimes.
Reach us at [email protected] . We read every message, and reply to many!
David Gelles reports on climate change and leads The Times’s Climate Forward newsletter and events series . More about David Gelles
Severe solar storm expected to supercharge northern lights on Friday
A severe solar storm is expected to supercharge the northern lights on Friday, with forecasts indicating that auroras could be seen as far south in the United States as Alabama.
The National Oceanic and Atmospheric Administration’s Space Weather Prediction Center said Thursday that a series of solar flares and eruptions from the sun could trigger severe geomagnetic storms and “spectacular displays of aurora” on Earth from Friday evening through the weekend.
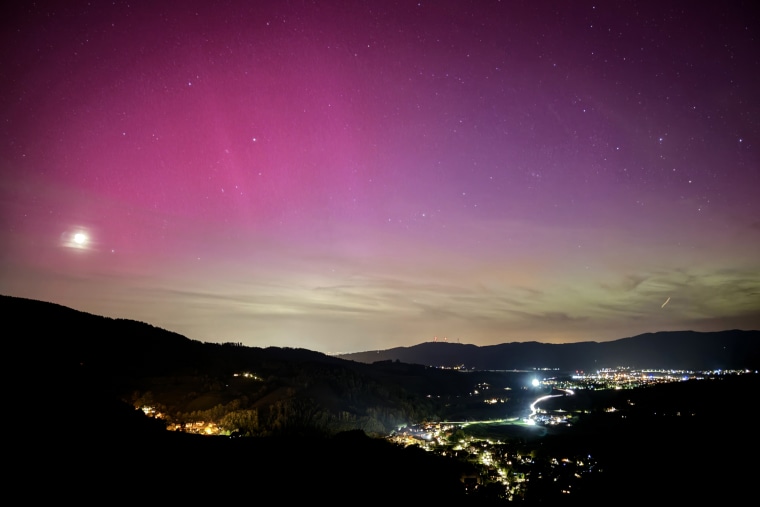
It was the first severe geomagnetic storm watch the agency has issued since 2005.
“We have a rare event on our hands,” said Shawn Dahl, a service coordinator at the Space Weather Prediction Center in Boulder, Colorado. "We're a little concerned. We haven't seen this in a long time."
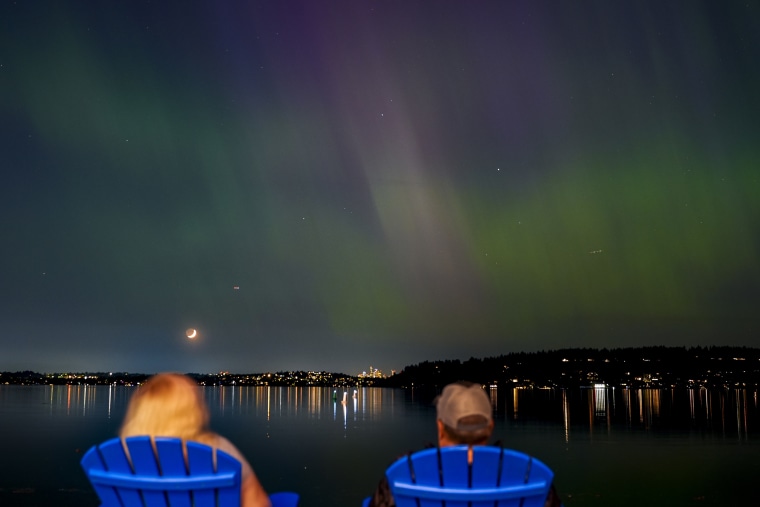
Because strong geomagnetic storms have the power to disrupt communications and power grids on Earth, as well as satellites in space, Dahl said satellite and grid operators have been notified to prepare.
He said forecasters predict the storm could arrive as soon as about 8 p.m. ET on Friday.
"We’re less certain on the timing of these events, because we’re talking about something for 93 million miles away," Dahl said, referring to the distance from the sun to the Earth.
A NASA spacecraft orbiting about 1 million miles from Earth, called the Advanced Composition Explorer, will help forecasters measure the solar wind and understand the timing and potential effects more precisely.
The northern lights , or aurora borealis, come from charged particles that spew from the sun during solar storms. The colorful displays are created when clouds of these energetic particles slam into Earth’s magnetic field and interact with the atoms and molecules in the planet’s upper atmosphere.
The northern lights typically light up the night sky at high latitudes, but during intense periods of solar activity, they can be spotted farther south than usual.
The Space Weather Prediction Center’s forecast said it’s possible that auroras on Friday night could be seen “as far south as Alabama and Northern California.”
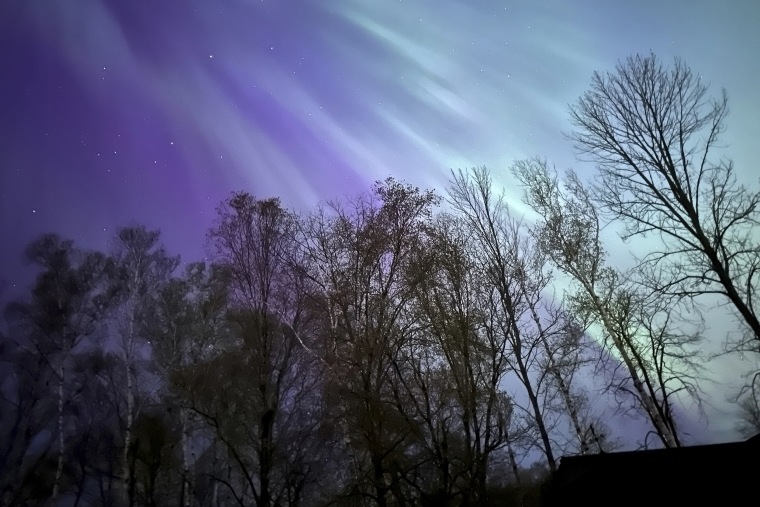
The agency maintains an aurora dashboard that provides short-term forecasts of the northern lights. If conditions are clear, auroras are best viewed from locations that are dark and far from city lights.
As night descended on parts of Australia and Europe, early photos began to emerge of dramatically colorful skies.
Dahl said smartphones might even be able to capture imagery of the aurora at southern locations where the human eye can't see anything unusual.
According to the Space Weather Prediction Center, several “moderate to strong” solar flares have been detected since Wednesday morning. Solar flares unleash clouds of plasma and charged particles, called coronal mass ejections, into space. At least five flares and their associated coronal mass ejections appear to be directed at Earth, the center said.
“Additional solar eruptions could cause geomagnetic storm conditions to persist through the weekend,” it said in a statement.
When directed at Earth, this geomagnetic and solar radiation can induce currents on high-voltage transmission lines and cause problems for transformers on the power grid.
One of the most damaging geomagnetic storms occurred in 1989, when roughly 6 million people in Montreal, Canada, lost power for nine hours, according to NASA . Some parts of the northeastern U.S. and Sweden were also affected in that event.
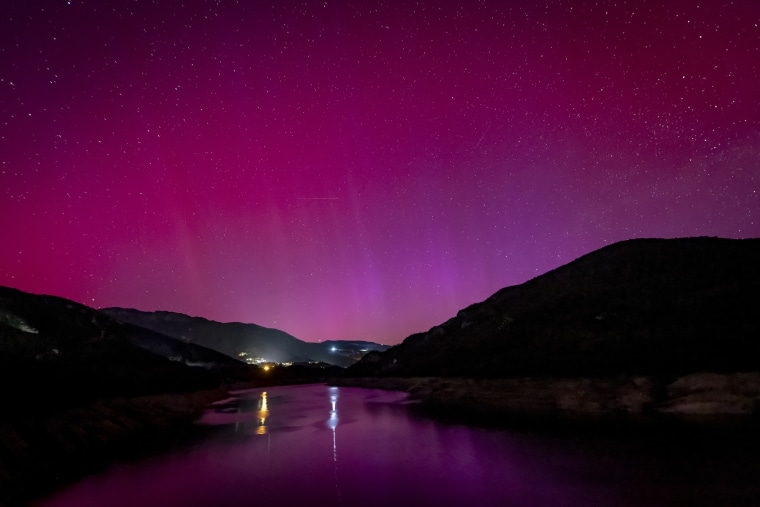
In 2002, a coronal mass ejection knocked out 38 commercial satellites .
The sun goes through 11-year cycles from minimum to maximum activity. The current cycle, which began in late 2019, is predicted to peak with maximum activity in July 2025 , according to NOAA and NASA forecasts.

Denise Chow is a reporter for NBC News Science focused on general science and climate change.
Evan Bush is a science reporter for NBC News. He can be reached at [email protected].
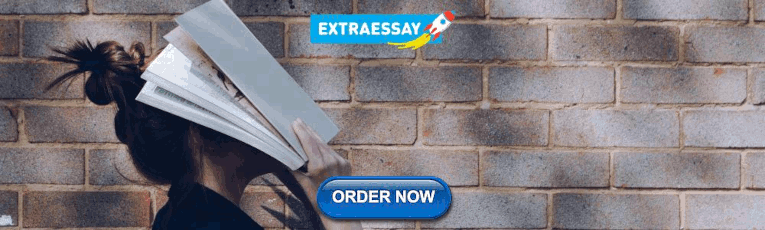
IMAGES
VIDEO
COMMENTS
Volcanic regions are also an excellent source of geothermal heat for both electricity and district heating, and of hydroelectric energy from streams. Figure 7.6.3 provides an overview of some of the ways that humans interact with volcanoes, and some of the risks associated with living nearby. Figure 7.6.3 Some of the Ways that Humans Interact ...
Volcanic eruptions are awe-inspiring natural phenomena that have fascinated and perplexed humanity throughout history. These explosive events, driven by the Earth's internal processes, shape landscapes and impact ecosystems in profound ways. Understanding the causes of volcanic eruptions is crucial for both scientific exploration and mitigating potential hazards associated with volcanic activity.
The 2008 eruption of Chaitén in Chile produced an ash cloud that blew 1,000 kilometers (620 miles) across Patagonia to Argentina, reaching both the Atlantic and Pacific Coasts. Volcanic ash deposits tend to be thicker and have larger particles closer to the eruption site. As distance from the volcano increases, the deposit tends to thin out.
FIGURE 1.1 NASA Ozone Monitoring Instrument observations of the SO 2 cloud produced by the August 7, 2008, eruption of Kasatochi (Aleutian Islands, Alaska) drifting over the lower 48 states and Canada on August 15, 2008. Satellite observations such as these are crucial for mitigating aviation hazards due to drifting volcanic clouds and for assessing the impact of volcanic eruptions on Earth ...
The second argument is the impact of volcanic er uption on climate. It explains the effects of volcanic. dust, Sulphur dioxide, and greenhouse gases, these three main volcanic substances that ...
Volcanic and sedimentary rocks, along with signals from deposited sulphate in ice cores, hold clues about how eruptions have altered conditions across our planet. The impacts can be temporary or ...
The gases and dust particles thrown into the atmosphere during large volcanic eruptions can influence climate. Particles spewed from volcanoes, like dust and ash, can cause temporary cooling by shading incoming solar radiation if the particles were launched high enough into the atmosphere. The cooling effect can last for months to years ...
A volcano is essentially an opening or a vent through which this magma and the dissolved gases it contains are discharged. Although there are several factors triggering a volcanic eruption, three ...
Globally, over a billion people are estimated to live within 100 km of an active volcano (Freire et al. 2019).Volcanic eruptions may cause injuries and fatalities via a range of hazardous phenomena (e.g., pyroclastic density currents, ballistics, lahars, lava flows, and localized accumulations or flows of asphyxiant gases such as CO 2 and H 2 S), affecting communities within tens of kilometers ...
Virtually all of this volcanic activity occurs underwater. In a few places the oceanic ridges are sufficiently elevated above the deep seafloor that they emerge from the ocean, and subaerial volcanism occurs. Iceland is the best-known example. The magmas that are erupted along the oceanic ridges are basaltic in composition.
Volcanic eruptions are among the most stunning phenomena in the natural world. Volcanoes erupt because of the way heat moves beneath Earth 's surface. Heat is conveyed from the planet's interior to its surface largely by convection —the transfer of heat by movement of a heated fluid. In this case, the fluid is magma —molten or partially ...
A volcano is an opening in a planet or moon's crust through which molten rock, hot gases, and other materials erupt. Volcanoes often form a hill or mountain as layers of rock and ash build up from repeated eruptions. Volcanoes are classified as active, dormant, or extinct. Active volcanoes have a recent history of eruptions; they are likely ...
Volcanoes are an inescapable part of our planet's geologic makeup, and their eruptions can have devastating consequences for the environment. In this essay, I will explore the myriad ways in which volcanoes affect the environment, including air pollution, climate change, and the creation of new landmasses.
A volcanic eruption is an eruption of molten rock, hot rock fragments, and hot gases through a volcano, which is a vent in a planet's or satellite's crust. Volcanic eruptions can cause disastrous loss of life and property. Volcanic eruptions play a role in climate change, with expelled gases such as carbon dioxide contributing to global warming, while ash, dust, and gases can drive global ...
When volcanoes erupt they can spew hot, dangerous gases, ash, lava and rock that can cause disastrous loss of life and property, especially in heavily populated areas. Volcanic activities and wildfires affected 6.2 million people and caused nearly 2400 deaths between 1998-2017. There are different types of volcanic eruptive events, including:
The new science of volcanoes harnesses AI, satellites and gas sensors to forecast eruptions. Although the cooling effects of sulfate aerosols in the stratosphere might counteract warming from ...
View Media Details. Volcanic gases react with the atmosphere in various ways; the conversion of sulfur dioxide (SO2) to sulfuric acid (H2SO4has the most significant impact on climate. Volcanoes can impact climate change. During major explosive eruptions huge amounts of volcanic gas, aerosol droplets, and ash are injected into the stratosphere.
When the volume of the bubbles formed is about 75%, the magma breaks into pyroclasts and bursts out. The three main causes of volcanic eruptions are: The buoyancy of the magma. Pressure from the exsolved gases in the magma. Increase in pressure on the chamber lid. Hope you are familiar with why volcanoes erupt and the cause of the volcanic ...
Volcanic Effects. Volcanoes affect people in many ways, some are good, some are not. Some of the bad ways are that houses, buildings, roads, and fields can get covered with ash. As long as you can get the ash off (especially if it is wet), your house may not collapse, but often the people leave because of the ash and are not around to ...
Causes. The volcano (Eyjafjallajökull) is situated on a divergent boundary and is a strato volcano that is built by many layers of lava and ash. The volcano was formed by water being converted into a gas. The explosion was caused by the common magma inside the volcano meeting with silica-rich magma. In contrast, the Honshu volcano is on the ...
Long Essay On Volcano For Children. Volcanic eruptions can happen through small cracks on the Earth's surface, fissures, and new landforms. Poisonous gases and debris get mixed with the lava released during these explosions. Here is a long essay for class 3 kids on volcanoes: Lava can come in different forms, and this is what makes volcanoes ...
Over time, volcanic ash and other ejected material can enrich the soil, making volcanic regions fertile for agriculture. The impact of volcanic eruptions on human civilization can be both destructive and beneficial. Throughout history, volcanic eruptions have caused loss of life and property, as well as disruptions to agriculture and trade.
Volcanoes emit large amounts of ash in the air. This volcanic ash and rain combine to form and cause flooding that can wash away roads, bridges, and can even flood nearby villages and cities, leaving many people homeless and destroying everything in its path. Soil, rock, and debris forming together can pile up like a flood and leave cities swamped.
However, the end of the fissure goes into the sea, which means the eruption can occur on the ocean floor, which would likely cause a large ash cloud. Volcanic eruptions also pose a serious hazard ...
(The exceptions, in 1963 and 1991, coincided with giant volcanic eruptions that can drive down temperatures as soot and rock particles produce a shading effect.)
The world is making steady progress in ramping up renewables. And that's a real cause for optimism. "The notion of a 'doomer camp' is overstated," Leiserowitz said. "The larger ...
Energy consumption worldwide has undergone a recent shift, with hydrocarbons, coal, and new energy sources taking center stage. However, fossil fuels face criticism due to their negative impacts on the environment through air pollution. Geothermal energy is a clean, renewable, and eco-friendly alternative that can be harnessed for power generation. The Lunayyir volcanic field is located in the ...
The National Oceanic and Atmospheric Administration's Space Weather Prediction Center said Thursday that a series of solar flares and eruptions from the sun could trigger severe geomagnetic ...