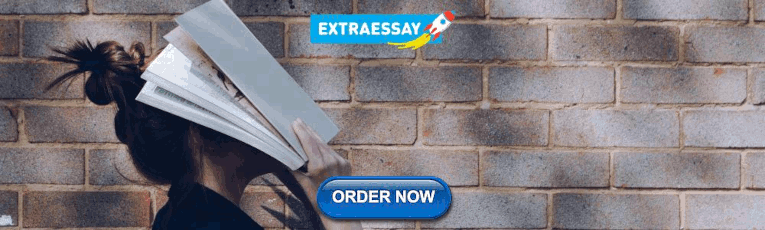
New Comprehensive Review Examines Potential Harms of COVID-19 Vaccination and Intramuscular Vaccination
WASHINGTON — A new report from the National Academies of Sciences, Engineering, and Medicine reviews evidence for 19 potential harms of the COVID-19 vaccines, and for nine potential shoulder injuries from intramuscular administration of vaccines more broadly. The committee that conducted the review identified sufficient evidence to draw 20 conclusions about whether these vaccines could cause specific harms, and drew 65 conclusions where it did not find enough evidence to establish, accept, or reject a causal relationship .
The Health Resources and Services Administration requested that the National Academies convene a committee to review the evidence regarding specific potential harms related to the COVID-19 vaccines used in the United States, for which HRSA had received claims for compensation under its Countermeasures Injury Compensation Program (CICP).
As part of “Operation Warp Speed,” a rapid response by the federal government to speed vaccine development during the COVID-19 pandemic, four vaccines were developed and authorized for emergency use in the U.S., with some now fully approved by the Food and Drug Administration. COVID-19 vaccines are estimated to have prevented 14.4 million deaths worldwide in the first year they were available.
COVID-19 Vaccines and Possible Harms
The report concludes that two messenger ribonucleic acid (mRNA) vaccines, manufactured by Pfizer-BioNTech and Moderna, can cause myocarditis — inflammation of the heart muscle. Evidence suggests the two mRNA vaccines do not cause infertility, Guillain-Barré syndrome, Bell’s palsy, thrombosis with thrombocytopenia syndrome (TTS), or myocardial infarction (heart attack). Evidence also suggests the Pfizer-BioNTech vaccine does not cause ischemic stroke.
Although there are a limited number of published studies on the Janssen vaccine, reflecting its limited use in the U.S., evidence suggests this vaccine may cause TTS and Guillain-Barré syndrome.
The report says that for all conclusions that do not establish a causal link, future studies that estimate effects more precisely or that better minimize bias and confounding variables could lead to a different finding.
“Despite a large body of evidence from extraordinary efforts by investigators around the world, our committee found that in many cases, if not most, evidence was insufficient to accept or reject causality for a particular potential harm from a specific COVID-19 vaccine,” said committee vice chair Anne Bass, professor of clinical medicine, Weill Cornell Medicine, and a rheumatologist at the Hospital for Special Surgery and New York Presbyterian Hospital. “In other cases, we did find sufficient evidence to favor rejection, favor acceptance, or establish causality. It is important to note, however, that identifying a harm does not mean that it occurs frequently. Harms associated with vaccines are rare.”
Shoulder Injuries and Possible Harms
HRSA also requested that the committee review the evidence regarding any vaccine administration — not specifically COVID-19 vaccines — and shoulder injuries , to help its National Vaccine Injury Compensation Program (VICP) better understand whether vaccination can cause very specific types of shoulder injuries or a more general syndrome that it designated as “Shoulder Injuries Related to Vaccine Administration.” The report says that evidence shows vaccination may cause four specific shoulder injuries: acute subacromial/subdeltoid bursitis caused by direct injection of a vaccine into the bursa; acute rotator cuff tendinopathy caused by direct injection into or adjacent to a tendon; bone injury caused by direct injection into or adjacent to bone; and axial or radial nerve injury due to direct injection into or adjacent to the nerve. In addition, evidence suggests that intramuscular vaccine administration does not cause chronic rotator cuff disease.
Evidence in Children
Because potential vaccine-associated harms may differ in children and adults, the committee conducted an in-depth review of literature on adverse events related to COVID-19 vaccines in children under 18, and found insufficient evidence to make conclusions specific to children. At the time of the committee’s review, data on children were only available for the Pfizer-BioNTech and Moderna vaccines, due to later authorization of COVID-19 vaccines for emergency use in children, and decreased uptake of COVID-19 vaccines in children, particularly those younger than 11. Ongoing and future pharmacovigilance and epidemiology studies should produce more definitive data on the risk and relative incidence of harms of COVID-19 vaccines in children.
About This Study
The report says all conclusions must be assessed in the context of the established harms of COVID-19 infection and the well-documented benefits of COVID-19 vaccines in preventing infection. The committee was not tasked with estimating the magnitude or strength of associations between vaccinations and outcomes, and did not offer conclusions regarding specific patient cases or whether VICP or CICP should award compensation in individual cases or in general.
“Given that the studies we reviewed were performed shortly after vaccines were available, the information in this report is a snapshot in time, and new vaccines will be developed and more research conducted,” said George Isham, senior fellow, HealthPartners Institute, and chair of the committee that wrote the report. “For example, the evidence does not address the real-world use of the COVID-19 vaccines in which many individuals received a ‘mix and match’ sequence of them. Many people vaccinated for COVID-19 also received other vaccines, such as influenza, simultaneously.”
The study — undertaken by the Committee to Review Relevant Literature Regarding Adverse Effects Associated with Vaccines — was sponsored by the Health Resources and Services Administration of the U.S. Department of Health and Human Services.
The National Academies of Sciences, Engineering, and Medicine are private, nonprofit institutions that provide independent, objective analysis and advice to the nation to solve complex problems and inform public policy decisions related to science, engineering, and medicine. They operate under an 1863 congressional charter to the National Academy of Sciences, signed by President Lincoln.
Dana Korsen, Director of Media Relations Megan Lowry, Media Relations Manager Office of News and Public Information 202-334-2138; e-mail [email protected]
Featured Publication
Evidence Review of the Adverse Effects of COVID-19 Vaccination and Intramuscular Vaccine Administration
Vaccines are a public health success story, as they have prevented or lessened the effects of many infectious diseases. To address concerns around potential vaccine injuries, the Health Resources and Services Administration (HRSA) administers the Vaccine Injury Compensation Program (VICP) and the Countermeasures Injury Compensation Program (CICP), which provide compensation to those who assert that they were injured by routine vaccines or medical countermeasures, respectively. The National Academies of Sciences, Engineering, and Medicine have contributed to the scientific basis for VICP compensation decisions for decades.
HRSA asked the National Academies to convene an expert committee to review the epidemiological, clinical, and biological evidence about the relationship between COVID-19 vaccines and specific adverse events, as well as intramuscular administration of vaccines and shoulder injuries. This report outlines the committee findings and conclusions.
Read Full Description
- Digital Resource: Evidence Review of the Adverse Effects of COVID-19 Vaccination
- Digital Resource: Evidence Review of Shoulder Injuries from Intramuscular Administration of Vaccines
- Press Release
Recent News
NAS Launches Science and Innovation Fund for Ukraine
Science Academies Issue Statements to Inform G7 Talks
Supporting Family Caregivers in STEMM
A Vision for High-Quality Preschool for All
- Load More...
- Scoping Review
- Open access
- Published: 14 November 2021
Effectiveness and safety of SARS-CoV-2 vaccine in real-world studies: a systematic review and meta-analysis
- Qiao Liu 1 na1 ,
- Chenyuan Qin 1 , 2 na1 ,
- Min Liu 1 &
- Jue Liu ORCID: orcid.org/0000-0002-1938-9365 1 , 2
Infectious Diseases of Poverty volume 10 , Article number: 132 ( 2021 ) Cite this article
56k Accesses
204 Citations
372 Altmetric
Metrics details
To date, coronavirus disease 2019 (COVID-19) becomes increasingly fierce due to the emergence of variants. Rapid herd immunity through vaccination is needed to block the mutation and prevent the emergence of variants that can completely escape the immune surveillance. We aimed to systematically evaluate the effectiveness and safety of COVID-19 vaccines in the real world and to establish a reliable evidence-based basis for the actual protective effect of the COVID-19 vaccines, especially in the ensuing waves of infections dominated by variants.
We searched PubMed, Embase and Web of Science from inception to July 22, 2021. Observational studies that examined the effectiveness and safety of SARS-CoV-2 vaccines among people vaccinated were included. Random-effects or fixed-effects models were used to estimate the pooled vaccine effectiveness (VE) and incidence rate of adverse events after vaccination, and their 95% confidence intervals ( CI ).
A total of 58 studies (32 studies for vaccine effectiveness and 26 studies for vaccine safety) were included. A single dose of vaccines was 41% (95% CI : 28–54%) effective at preventing SARS-CoV-2 infections, 52% (31–73%) for symptomatic COVID-19, 66% (50–81%) for hospitalization, 45% (42–49%) for Intensive Care Unit (ICU) admissions, and 53% (15–91%) for COVID-19-related death; and two doses were 85% (81–89%) effective at preventing SARS-CoV-2 infections, 97% (97–98%) for symptomatic COVID-19, 93% (89–96%) for hospitalization, 96% (93–98%) for ICU admissions, and 95% (92–98%) effective for COVID-19-related death, respectively. The pooled VE was 85% (80–91%) for the prevention of Alpha variant of SARS-CoV-2 infections, 75% (71–79%) for the Beta variant, 54% (35–74%) for the Gamma variant, and 74% (62–85%) for the Delta variant. The overall pooled incidence rate was 1.5% (1.4–1.6%) for adverse events, 0.4 (0.2–0.5) per 10 000 for severe adverse events, and 0.1 (0.1–0.2) per 10 000 for death after vaccination.
Conclusions
SARS-CoV-2 vaccines have reassuring safety and could effectively reduce the death, severe cases, symptomatic cases, and infections resulting from SARS-CoV-2 across the world. In the context of global pandemic and the continuous emergence of SARS-CoV-2 variants, accelerating vaccination and improving vaccination coverage is still the most important and urgent matter, and it is also the final means to end the pandemic.
Graphical Abstract
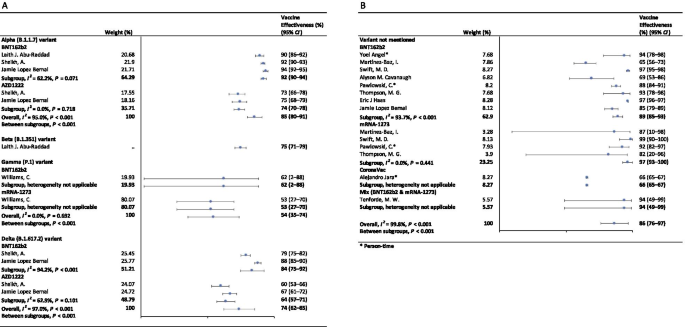
Since its outbreak, coronavirus disease 2019 (COVID-19) has spread rapidly, with a sharp rise in the accumulative number of infections worldwide. As of August 8, 2021, COVID-19 has already killed more than 4.2 million people and more than 203 million people were infected [ 1 ]. Given its alarming-spreading speed and the high cost of completely relying on non-pharmaceutical measures, we urgently need safe and effective vaccines to cover susceptible populations and restore people’s lives into the original [ 2 ].
According to global statistics, as of August 2, 2021, there are 326 candidate vaccines, 103 of which are in clinical trials, and 19 vaccines have been put into normal use, including 8 inactivated vaccines and 5 protein subunit vaccines, 2 RNA vaccines, as well as 4 non-replicating viral vector vaccines [ 3 ]. Our World in Data simultaneously reported that 27.3% of the world population has received at least one dose of a COVID-19 vaccine, and 13.8% is fully vaccinated [ 4 ].
To date, COVID-19 become increasingly fierce due to the emergence of variants [ 5 , 6 , 7 ]. Rapid herd immunity through vaccination is needed to block the mutation and prevent the emergence of variants that can completely escape the immune surveillance [ 6 , 8 ]. Several reviews systematically evaluated the effectiveness and/or safety of the three mainstream vaccines on the market (inactivated virus vaccines, RNA vaccines and viral vector vaccines) based on random clinical trials (RCT) yet [ 9 , 10 , 11 , 12 , 13 ].
In general, RNA vaccines are the most effective, followed by viral vector vaccines and inactivated virus vaccines [ 10 , 11 , 12 , 13 ]. The current safety of COVID-19 vaccines is acceptable for mass vaccination, but long-term monitoring of vaccine safety is needed, especially in older people with underlying conditions [ 9 , 10 , 11 , 12 , 13 ]. Inactivated vaccines had the lowest incidence of adverse events and the safety comparisons between mRNA vaccines and viral vectors were controversial [ 9 , 10 ].
RCTs usually conduct under a very demanding research circumstance, and tend to be highly consistent and limited in terms of population characteristics and experimental conditions. Actually, real-world studies differ significantly from RCTs in terms of study conditions and mass vaccination in real world requires taking into account factors, which are far more complex, such as widely heterogeneous populations, vaccine supply, willingness, medical accessibility, etc. Therefore, the real safety and effectiveness of vaccines turn out to be a major concern of international community. The results of a mass vaccination of CoronaVac in Chile demonstrated a protective effectiveness of 65.9% against the onset of COVID-19 after complete vaccination procedures [ 14 ], while the outcomes of phase 3 trials in Brazil and Turkey were 50.7% and 91.3%, reported on Sinovac’s website [ 14 ]. As for the Delta variant, the British claimed 88% protection after two doses of BNT162b2, compared with 67% for AZD1222 [ 15 ]. What is surprising is that the protection of BNT162b2 against infection in Israel is only 39% [ 16 ]. Several studies reported the effectiveness and safety of the COVID-19 vaccine in the real world recently, but the results remain controversial [ 17 , 18 , 19 , 20 ]. A comprehensive meta-analysis based upon the real-world studies is still in an urgent demand, especially for evaluating the effect of vaccines on variation strains. In the present study, we aimed to systematically evaluate the effectiveness and safety of the COVID-19 vaccine in the real world and to establish a reliable evidence-based basis for the actual protective effect of the COVID-19 vaccines, especially in the ensuing waves of infections dominated by variants.
Search strategy and selection criteria
Our methods were described in detail in our published protocol [PROSPERO (Prospective register of systematic reviews) registration, CRD42021267110]. We searched eligible studies published by 22 July 2021, from three databases including PubMed, Embase and Web of Science by the following search terms: (effectiveness OR safety) AND (COVID-19 OR coronavirus OR SARS-CoV-2) AND (vaccine OR vaccination). We used EndNoteX9.0 (Thomson ResearchSoft, Stanford, USA) to manage records, screen and exclude duplicates. This study was strictly performed according to the Preferred Reporting Items for Systematic Reviews and Meta-Analyses (PRISMA).
We included observational studies that examined the effectiveness and safety of severe acute respiratory syndrome coronavirus 2 (SARS-CoV-2) vaccines among people vaccinated with SARS-CoV-2 vaccines. The following studies were excluded: (1) irrelevant to the subject of the meta-analysis, such as studies that did not use SARS-CoV-2 vaccination as the exposure; (2) insufficient data to calculate the rate for the prevention of COVID-19, the prevention of hospitalization, the prevention of admission to the ICU, the prevention of COVID-19-related death, or adverse events after vaccination; (3) duplicate studies or overlapping participants; (4) RCT studies, reviews, editorials, conference papers, case reports or animal experiments; and (5) studies that did not clarify the identification of COVID-19.
Studies were identified by two investigators (LQ and QCY) independently following the criteria above, while discrepancies reconciled by a third investigator (LJ).
Data extraction and quality assessment
The primary outcome was the effectiveness of SARS-CoV-2 vaccines. The following data were extracted independently by two investigators (LQ and QCY) from the selected studies: (1) basic information of the studies, including first author, publication year and study design; (2) characteristics of the study population, including sample sizes, age groups, setting or locations; (3) kinds of the SARS-CoV-2 vaccines; (4) outcomes for the effectiveness of SARS-CoV-2 vaccines: the number of laboratory-confirmed COVID-19, hospitalization for COVID-19, admission to the ICU for COVID-19, and COVID-19-related death; and (5) outcomes for the safety of SARS-CoV-2 vaccines: the number of adverse events after vaccination.
We evaluated the risk of bias using the Newcastle–Ottawa quality assessment scale for cohort studies and case–control studies [ 21 ]. and assess the methodological quality using the checklist recommended by Agency for Healthcare Research and Quality (AHRQ) [ 22 ]. Cohort studies and case–control studies were classified as having low (≥ 7 stars), moderate (5–6 stars), and high risk of bias (≤ 4 stars) with an overall quality score of 9 stars. For cross-sectional studies, we assigned each item of the AHRQ checklist a score of 1 (answered “yes”) or 0 (answered “no” or “unclear”), and summarized scores across items to generate an overall quality score that ranged from 0 to 11. Low, moderate, and high risk of bias were identified as having a score of 8–11, 4–7 and 0–3, respectively.
Two investigators (LQ and QCY) independently assessed study quality, with disagreements resolved by a third investigator (LJ).
Data synthesis and statistical analysis
We performed a meta-analysis to pool data from included studies and assess the effectiveness and safety of SARS-CoV-2 vaccines by clinical outcomes (rates of the prevention of COVID-19, the prevention of hospitalization, the prevention of admission to the ICU, the prevention of COVID-19-related death, and adverse events after vaccination). Random-effects or fixed-effects models were used to pool the rates and adjusted estimates across studies separately, based on the heterogeneity between estimates ( I 2 ). Fixed-effects models were used if I 2 ≤ 50%, which represented low to moderate heterogeneity and random-effects models were used if I 2 > 50%, representing substantial heterogeneity.
We conducted subgroup analyses to investigate the possible sources of heterogeneity by using vaccine kinds, vaccination status, sample size, and study population as grouping variables. We used the Q test to conduct subgroup comparisons and variables were considered significant between subgroups if the subgroup difference P value was less than 0.05. Publication bias was assessed by funnel plot and Egger’s regression test. We analyzed data using Stata version 16.0 (StataCorp, Texas, USA).
A total of 4844 records were searched from the three databases. 2484 duplicates were excluded. After reading titles and abstracts, we excluded 2264 reviews, RCT studies, duplicates and other studies meeting our exclude criteria. Among the 96 studies under full-text review, 41 studies were excluded (Fig. 1 ). Ultimately, with three grey literatures included, this final meta-analysis comprised 58 eligible studies, including 32 studies [ 14 , 15 , 17 , 18 , 19 , 20 , 23 , 24 , 25 , 26 , 27 , 28 , 29 , 30 , 31 , 32 , 33 , 34 , 35 , 36 , 37 , 38 , 39 , 40 , 41 , 42 , 43 , 44 , 45 , 46 , 47 , 48 ] for vaccine effectiveness and 26 studies [ 49 , 50 , 51 , 52 , 53 , 54 , 55 , 56 , 57 , 58 , 59 , 60 , 61 , 62 , 63 , 64 , 65 , 66 , 67 , 68 , 69 , 70 , 71 , 72 , 73 , 74 ] for vaccine safety. Characteristics of included studies are showed in Additional file 1 : Table S1, Additional file 2 : Table S2. The risk of bias of all studies we included was moderate or low.
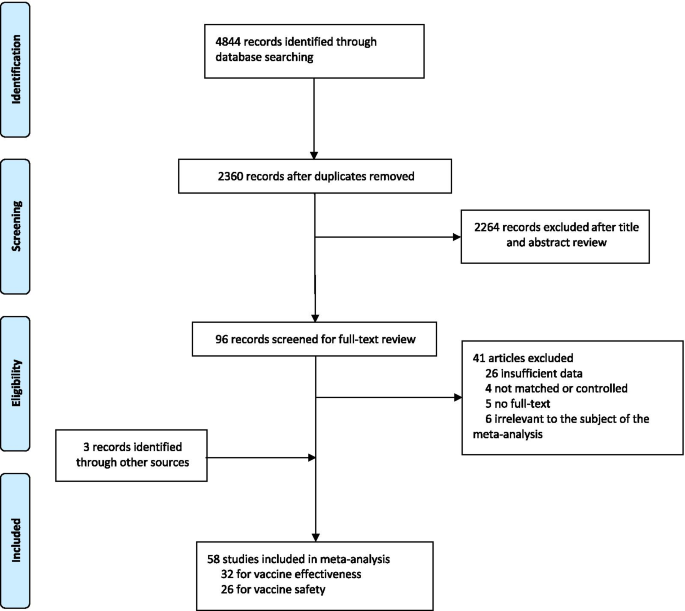
Flowchart of the study selection
Vaccine effectiveness for different clinical outcomes of COVID-19
We separately reported the vaccine effectiveness (VE) by the first and second dose of vaccines, and conducted subgroup analysis by the days after the first or second dose (< 7 days, ≥ 7 days, ≥ 14 days, and ≥ 21 days; studies with no specific days were classified as 1 dose, 2 dose or ≥ 1 dose).
For the first dose of SARS-CoV-2 vaccines, the pooled VE was 41% (95% CI : 28–54%) for the prevention of SARS-CoV-2 infection, 52% (95% CI : 31–73%) for the prevention of symptomatic COVID-19, 66% (95% CI : 50–81%) for the prevention of hospital admissions, 45% (95% CI : 42–49%) for the prevention of ICU admissions, and 53% (95% CI : 15–91%) for the prevention of COVID-19-related death (Table 1 ). The subgroup, ≥ 21 days after the first dose, was found to have the highest VE in each clinical outcome of COVID-19, regardless of ≥ 1 dose group (Table 1 ).
For the second dose of SARS-CoV-2 vaccines, the pooled VE was 85% (95% CI : 81–89%) for the prevention of SARS-CoV-2 infection, 97% (95% CI : 97–98%) for the prevention of symptomatic COVID-19, 93% (95% CI: 89–96%) for the prevention of hospital admissions, 96% (95% CI : 93–98%) for the prevention of ICU admissions, and 95% (95% CI : 92–98%) for the prevention of COVID-19-related death (Table 1 ). VE was 94% (95% CI : 78–98%) in ≥ 21 days after the second dose for the prevention of SARS-CoV-2 infection, higher than other subgroups, regardless of 2 dose group (Table 1 ). For the prevention of symptomatic COVID-19, VE was also relatively higher in 21 days after the second dose (99%, 95% CI : 94–100%). Subgroups showed no statistically significant differences in the prevention of hospital admissions, ICU admissions and COVID-19-related death (subgroup difference P values were 0.991, 0.414, and 0.851, respectively).
Vaccine effectiveness for different variants of SARS-CoV-2 in fully vaccinated people
In the fully vaccinated groups (over 14 days after the second dose), the pooled VE was 85% (95% CI: 80–91%) for the prevention of Alpha variant of SARS-CoV-2 infection, 54% (95% CI : 35–74%) for the Gamma variant, and 74% (95% CI : 62–85%) for the Delta variant. There was only one study [ 23 ] focused on the Beta variant, which showed the VE was 75% (95% CI : 71–79%) for the prevention of the Beta variant of SARS-CoV-2 infection. BNT162b2 vaccine had the highest VE in each variant group; 92% (95% CI : 90–94%) for the Alpha variant, 62% (95% CI : 2–88%) for the Gamma variant, and 84% (95% CI : 75–92%) for the Delta variant (Fig. 2 ).
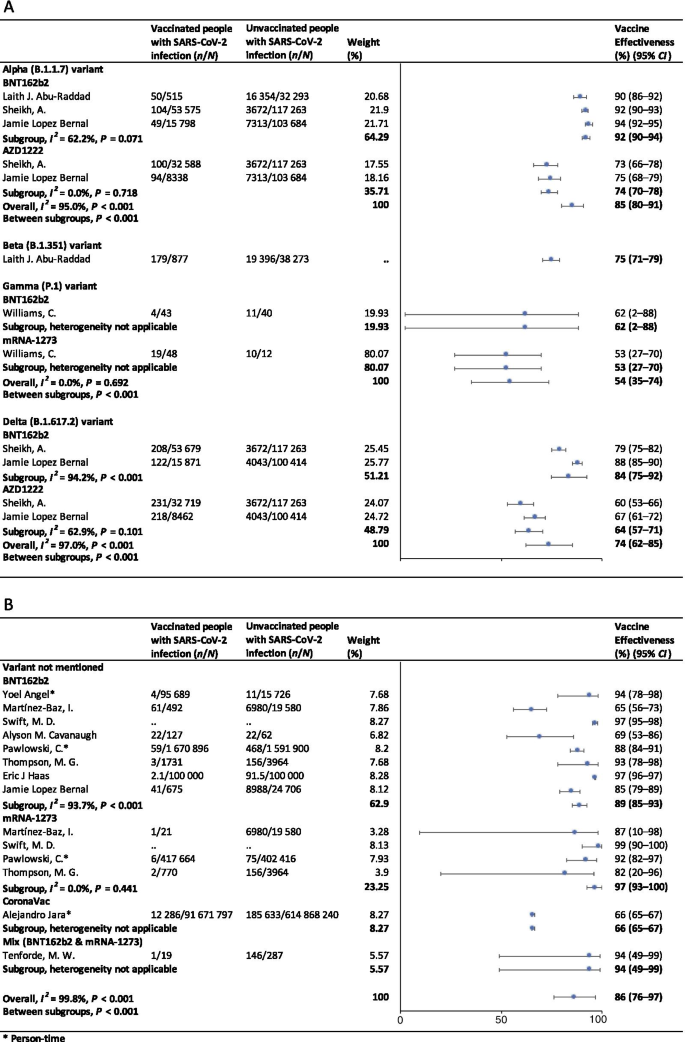
Forest plots for the vaccine effectiveness of SARS-CoV-2 vaccines in fully vaccinated populations. A Vaccine effectiveness against SARS-CoV-2 variants; B Vaccine effectiveness against SARS-CoV-2 with variants not mentioned. SARS-CoV-2 severe acute respiratory syndrome coronavirus 2, COVID-19 coronavirus disease 2019, CI confidence interval
For studies which had not mentioned the variant of SARS-CoV-2, the pooled VE was 86% (95% CI: 76–97%) for the prevention of SARS-CoV-2 infection in fully vaccinated people. mRNA-1273 vaccine had the highest pooled VE (97%, 95% CI: 93–100%, Fig. 2 ).
Safety of SARS-CoV-2 vaccines
As Table 2 showed, the incidence rate of adverse events varied widely among different studies. We conducted subgroup analysis by study population (general population, patients and healthcare workers), vaccine type (BNT162b2, mRNA-1273, CoronaVac, and et al.), and population size (< 1000, 1000–10 000, 10 000–100 000, and > 100 000). The overall pooled incidence rate was 1.5% (95% CI : 1.4–1.6%) for adverse events, 0.4 (95% CI : 0.2–0.5) per 10 000 for severe adverse events, and 0.1 (95% CI : 0.1–0.2) per 10 000 for death after vaccination. Incidence rate of adverse events was higher in healthcare workers (53.2%, 95% CI : 28.4–77.9%), AZD1222 vaccine group (79.6%, 95% CI : 60.8–98.3%), and < 1000 population size group (57.6%, 95% CI : 47.9–67.4%). Incidence rate of sever adverse events was higher in healthcare workers (127.2, 95% CI : 62.7–191.8, per 10 000), Gam-COVID-Vac vaccine group (175.7, 95% CI : 77.2–274.2, per 10 000), and 1000–10 000 population size group (336.6, 95% CI : 41.4–631.8, per 10 000). Incidence rate of death after vaccination was higher in patients (7.6, 95% CI : 0.0–32.2, per 10 000), BNT162b2 vaccine group (29.8, 95% CI : 0.0–71.2, per 10 000), and < 1000 population size group (29.8, 95% CI : 0.0–71.2, per 10 000). Subgroups of general population, vaccine type not mentioned, and > 100 000 population size had the lowest incidence rate of adverse events, severe adverse events, and death after vaccination.
Sensitivity analysis and publication bias
In the sensitivity analyses, VE for SARS-CoV-2 infections, symptomatic COVID-19 and COVID-19-related death got relatively lower when omitting over a single dose group of Maria et al.’s work [ 33 ]; when omitting ≥ 14 days after the first dose group and ≥ 14 days after the second dose group of Alejandro et al.’s work [ 14 ], VE for SARS-CoV-2 infections, hospitalization, ICU admission and COVID-19-related death got relatively higher; and VE for all clinical status of COVID-19 became lower when omitting ≥ 14 days after the second dose group of Eric et al.’s work [ 34 ]. Incidence rate of adverse events and severe adverse events got relatively higher when omitting China CDC’s data [ 74 ]. P values of Egger’s regression test for all the meta-analysis were more than 0.05, indicating that there might not be publication bias.
To our knowledge, this is a comprehensive systematic review and meta-analysis assessing the effectiveness and safety of SARS-CoV-2 vaccines based on real-world studies, reporting pooled VE for different variants of SARS-CoV-2 and incidence rate of adverse events. This meta-analysis comprised a total of 58 studies, including 32 studies for vaccine effectiveness and 26 studies for vaccine safety. We found that a single dose of SARS-CoV-2 vaccines was about 40–60% effective at preventing any clinical status of COVID-19 and that two doses were 85% or more effective. Although vaccines were not as effective against variants of SARS-CoV-2 as original virus, the vaccine effectiveness was still over 50% for fully vaccinated people. Normal adverse events were common, while the incidence of severe adverse events or even death was very low, providing reassurance to health care providers and to vaccine recipients and promote confidence in the safety of COVID-19 vaccines. Our findings strengthen and augment evidence from previous review [ 75 ], which confirmed the effectiveness of the BNT162b2 mRNA vaccine, and additionally reported the safety of SARS-CoV-2 vaccines, giving insight on the future of SARS-CoV-2 vaccine schedules.
Although most vaccines for the prevention of COVID-19 are two-dose vaccines, we found that the pooled VE of a single dose of SARS-CoV-2 vaccines was about 50%. Recent study showed that the T cell and antibody responses induced by a single dose of the BNT162b2 vaccine were comparable to those naturally infected with SARE-CoV-2 within weeks or months after infection [ 76 ]. Our findings could help to develop vaccination strategies under certain circumstances such as countries having a shortage of vaccines. In some countries, in order to administer the first dose to a larger population, the second dose was delayed for up to 12 weeks [ 77 ]. Some countries such as Canada had even decided to delay the second dose for 16 weeks [ 78 ]. However, due to a suboptimum immune response in those receiving only a single dose of a vaccine, such an approach had a chance to give rise to the emergence of variants of SARS-CoV-2 [ 79 ]. There remains a need for large clinical trials to assess the efficacy of a single-dose administration of two-dose vaccines and the risk of increasing the emergence of variants.
Two doses of SARS-CoV-2 vaccines were highly effective at preventing hospitalization, severe cases and deaths resulting from COVID-19, while the VE of different groups of days from the second vaccine dose showed no statistically significant differences. Our findings emphasized the importance of getting fully vaccinated, for the fact that most breakthrough infections were mild or asymptomatic. A recent study showed that the occurrence of breakthrough infections with SARS-CoV-2 in fully vaccinated populations was predictable with neutralizing antibody titers during the peri-infection period [ 80 ]. We also found getting fully vaccinated was at least 50% effective at preventing SARS-CoV-2 variants infections, despite reduced effectiveness compared with original virus; and BNT162b2 vaccine was found to have the highest VE in each variant group. Studies showed that the highly mutated variants were indicative of a form of rapid, multistage evolutionary jumps, which could preferentially occur in the milieu of partial immune control [ 81 , 82 ]. Therefore, immunocompromised patients should be prioritized for anti-COVID-19 immunization to mitigate persistent SARS-CoV-2 infections, during which multimutational SARS-CoV-2 variants could arise [ 83 ].
Recently, many countries, including Israel, the United States, China and the United Kingdom, have introduced a booster of COVID-19 vaccine, namely the third dose [ 84 , 85 , 86 , 87 ]. A study of Israel showed that among people vaccinated with BNT162b2 vaccine over 60 years, the risk of COVID-19 infection and severe illness in the non-booster group was 11.3 times (95% CI: 10.4–12.3) and 19.5 times (95% CI: 12.9–29.5) than the booster group, respectively [ 84 ]. Some studies have found that the third dose of Moderna, Pfizer-BioNTech, Oxford-AstraZeneca and Sinovac produced a spike in infection-blocking neutralizing antibodies when given a few months after the second dose [ 85 , 87 , 88 ]. In addition, the common adverse events associated with the third dose did not differ significantly from the symptoms of the first two doses, ranging from mild to moderate [ 85 ]. The overall incidence rate of local and systemic adverse events was 69% (57/97) and 20% (19/97) after receiving the third dose of BNT162b2 vaccine, respectively [ 88 ]. Results of a phase 3 clinical trial involving 306 people aged 18–55 years showed that adverse events after receiving a third dose of BNT162b2 vaccine (5–8 months after completion of two doses) were similar to those reported after receiving a second dose [ 85 ]. Based on V-safe, local reactions were more frequently after dose 3 (5323/6283; 84.7%) than dose 2 (5249/6283; 83.5%) among people who received 3 doses of Moderna. Systemic reactions were reported less frequently after dose 3 (4963/6283; 79.0%) than dose 2 (5105/6283; 81.3%) [ 86 ]. On August 4, WHO called for a halt to booster shots until at least the end of September to achieve an even distribution of the vaccine [ 89 ]. At this stage, the most important thing we should be thinking about is how to reach a global cover of people at risk with the first or second dose, rather than focusing on the third dose.
Based on real world studies, our results preliminarily showed that complete inoculation of COVID-19 vaccines was still effective against infection of variants, although the VE was generally diminished compared with the original virus. Particularly, the pooled VE was 54% (95% CI : 35–74%) for the Gamma variant, and 74% (95% CI : 62–85%) for the Delta variant. Since the wide spread of COVID-19, a number of variants have drawn extensive attention of international community, including Alpha variant (B.1.1.7), first identified in the United Kingdom; Beta variant (B.1.351) in South Africa; Gamma variant (P.1), initially appeared in Brazil; and the most infectious one to date, Delta variant (B.1.617.2) [ 90 ]. Israel recently reported a breakthrough infection of SARS-CoV-2, dominated by variant B.1.1.7 in a small number of fully vaccinated health care workers, raising concerns about the effectiveness of the original vaccine against those variants [ 80 ]. According to an observational cohort study in Qatar, VE of the BNT162b2 vaccine against the Alpha (B.1.1.7) and Beta (B.1.351) variants was 87% (95% CI : 81.8–90.7%) and 75.0% (95% CI : 70.5–7.9%), respectively [ 23 ]. Based on the National Immunization Management System of England, results from a recent real-world study of all the general population showed that the AZD1222 and BNT162b2 vaccines protected against symptomatic SARS-CoV-2 infection of Alpha variant with 74.5% (95% CI : 68.4–79.4%) and 93.7% (95% CI : 91.6–95.3%) [ 15 ]. In contrast, the VE against the Delta variant was 67.0% (95% CI : 61.3–71.8%) for two doses of AZD1222 vaccine and 88% (95% CI : 85.3–90.1%) for BNT162b2 vaccine [ 15 ].
In terms of adverse events after vaccination, the pooled incidence rate was very low, only 1.5% (95% CI : 1.4–1.6%). However, the prevalence of adverse events reported in large population (population size > 100 000) was much lower than that in small to medium population size. On the one hand, the vaccination population in the small to medium scale studies we included were mostly composed by health care workers, patients with specific diseases or the elderly. And these people are more concerned about their health and more sensitive to changes of themselves. But it remains to be proved whether patients or the elderly are more likely to have adverse events than the general. Mainstream vaccines currently on the market have maintained robust safety in specific populations such as cancer patients, organ transplant recipients, patients with rheumatic and musculoskeletal diseases, pregnant women and the elderly [ 54 , 91 , 92 , 93 , 94 ]. A prospective study by Tal Goshen-lag suggests that the safety of BNT162b2 vaccine in cancer patients is consistent with those previous reports [ 91 ]. In addition, the incidence rate of adverse events reported in the heart–lung transplant population is even lower than that in general population [ 95 ]. On the other hand, large scale studies at the national level are mostly based on national electronic health records or adverse event reporting systems, and it is likely that most mild or moderate symptoms are actually not reported.
Compared with the usual local adverse events (such as pain at the injection site, redness at the injection site, etc.) and normal systemic reactions (such as fatigue, myalgia, etc.), serious and life-threatening adverse events were rare due to our results. A meta-analysis based on RCTs only showed three cases of anaphylactic shock among 58 889 COVID-19 vaccine recipients and one in the placebo group [ 11 ]. The exact mechanisms underlying most of the adverse events are still unclear, accordingly we cannot establish a causal relation between severe adverse events and vaccination directly based on observational studies. In general, varying degrees of adverse events occur after different types of COVID-19 vaccination. Nevertheless, the benefits far outweigh the risks.
Our results showed the effectiveness and safety of different types of vaccines varied greatly. Regardless of SARS-CoV-2 variants, vaccine effectiveness varied from 66% (CoronaVac [ 14 ]) to 97% (mRNA-1273 [ 18 , 20 , 45 , 46 ]). The incidence rate of adverse events varied widely among different types of vaccines, which, however, could be explained by the sample size and population group of participants. BNT162b2, AZD1222, mRNA-1273 and CoronaVac were all found to have high vaccine efficacy and acceptable adverse-event profile in recent published studies [ 96 , 97 , 98 , 99 ]. A meta-analysis, focusing on the potential vaccine candidate which have reached to the phase 3 of clinical development, also found that although many of the vaccines caused more adverse events than the controls, most were mild, transient and manageable [ 100 ]. However, severe adverse events did occur, and there remains the need to implement a unified global surveillance system to monitor the adverse events of COVID-19 vaccines around the world [ 101 ]. A recent study employed a knowledge-based or rational strategy to perform a prioritization matrix of approved COVID-19 vaccines, and led to a scale with JANSSEN (Ad26.COV2.S) in the first place, and AZD1222, BNT162b2, and Sputnik V in second place, followed by BBIBP-CorV, CoronaVac and mRNA-1273 in third place [ 101 ]. Moreover, when deciding the priority of vaccines, the socioeconomic characteristics of each country should also be considered.
Our meta-analysis still has several limitations. First, we may include limited basic data on specific populations, as vaccination is slowly being promoted in populations under the age of 18 or over 60. Second, due to the limitation of the original real-world study, we did not conduct subgroup analysis based on more population characteristics, such as age. When analyzing the efficacy and safety of COVID-19 vaccine, we may have neglected the discussion on the heterogeneity from these sources. Third, most of the original studies only collected adverse events within 7 days after vaccination, which may limit the duration of follow-up for safety analysis.
Based on the real-world studies, SARS-CoV-2 vaccines have reassuring safety and could effectively reduce the death, severe cases, symptomatic cases, and infections resulting from SARS-CoV-2 across the world. In the context of global pandemic and the continuous emergence of SARS-CoV-2 variants, accelerating vaccination and improving vaccination coverage is still the most important and urgent matter, and it is also the final means to end the pandemic.
Availability of data and materials
All data generated or analyzed during this study are included in this published article and its additional information files.
Abbreviations
Coronavirus disease 2019
Severe Acute Respiratory Syndrome Coronavirus 2
Vaccine effectiveness
Confidence intervals
Intensive care unit
Random clinical trials
Preferred reporting items for systematic reviews and meta-analyses
COVID-19 Dashboard by the Center for Systems Science and Engineering (CSSE) at Johns Hopkins University (JHU). 2021. https://coronavirus.jhu.edu/map.html . Accessed 20 Aug 2021.
Barranco R, Rocca G, Molinelli A, Ventura F. Controversies and challenges of mass vaccination against SARS-CoV-2 in Italy: medico-legal perspectives and considerations. Healthcare (Basel). 2021. https://doi.org/10.3390/healthcare9091163 .
Article Google Scholar
COVID-19 vaccine tracker. 2021. https://vac-lshtm.shinyapps.io/ncov_vaccine_landscape/ . Accessed 20 Aug 2021.
Coronavirus (COVID-19) Vaccinations. 2021. https://ourworldindata.org/covid-vaccinations . Accessed 20 Aug 2021.
Kirby T. New variant of SARS-CoV-2 in UK causes surge of COVID-19. Lancet Respir Med. 2021;9(2):e20–1. https://doi.org/10.1016/s2213-2600(21)00005-9 .
Article CAS PubMed PubMed Central Google Scholar
Callaway E. Fast-spreading COVID variant can elude immune responses. Nature. 2021;589(7843):500–1. https://doi.org/10.1038/d41586-021-00121-z .
Article CAS PubMed Google Scholar
Reardon S. How the Delta variant achieves its ultrafast spread. Nature. 2021. https://doi.org/10.1038/d41586-021-01986-w .
Article PubMed Google Scholar
Li R, Liu J, Zhang H. The challenge of emerging SARS-CoV-2 mutants to vaccine development. J Genet Genomics. 2021;48(2):102–6. https://doi.org/10.1016/j.jgg.2021.03.001 .
Article PubMed PubMed Central Google Scholar
Chen M, Yuan Y, Zhou Y, Deng Z, Zhao J, Feng F, Zou H, Sun C. Safety of SARS-CoV-2 vaccines: a systematic review and meta-analysis of randomized controlled trials. Infect Dis Poverty. 2021;10(1):94. https://doi.org/10.1186/s40249-021-00878-5 .
Ling Y, Zhong J, Luo J. Safety and effectiveness of SARS-CoV-2 vaccines: a systematic review and meta-analysis. J Med Virol. 2021. https://doi.org/10.1002/jmv.27203 .
Pormohammad A, Zarei M, Ghorbani S, Mohammadi M, Razizadeh MH, Turner DL, Turner RJ. Efficacy and safety of COVID-19 vaccines: a systematic review and meta-analysis of randomized clinical trials. Vaccines (Basel). 2021. https://doi.org/10.3390/vaccines9050467 .
Sathian B, Asim M, Banerjee I, Roy B, Pizarro AB, Mancha MA, van Teijlingen ER, Kord-Varkaneh H, Mekkodathil AA, Subramanya SH, et al. Development and implementation of a potential coronavirus disease 2019 (COVID-19) vaccine: a systematic review and meta-analysis of vaccine clinical trials. Nepal J Epidemiol. 2021;11(1):959–82. https://doi.org/10.3126/nje.v11i1.36163 .
Yuan P, Ai P, Liu Y, Ai Z, Wang Y, Cao W, Xia X, Zheng JC. Safety, tolerability, and immunogenicity of COVID-19 vaccines: a systematic review and meta-analysis. medRxiv. 2020. https://doi.org/10.1101/2020.11.03.20224998 .
Jara A, Undurraga EA, González C, Paredes F, Fontecilla T, Jara G, Pizarro A, Acevedo J, Leo K, Leon F, et al. Effectiveness of an inactivated SARS-CoV-2 vaccine in Chile. N Engl J Med. 2021. https://doi.org/10.1056/NEJMoa2107715 .
Lopez Bernal J, Andrews N, Gower C, Gallagher E, Simmons R, Thelwall S, Stowe J, Tessier E, Groves N, Dabrera G, et al. Effectiveness of COVID-19 vaccines against the B.1.617.2 (Delta) variant. N Engl J Med. 2021. https://doi.org/10.1056/NEJMoa2108891 .
Israel says Pfizer Covid vaccine is just 39% effective as delta spreads, but still prevents severe illness. 2021. https://www.cnbc.com/2021/07/23/delta-variant-pfizer-covid-vaccine-39percent-effective-in-israel-prevents-severe-illness.html . Accessed 20 Aug 2021.
Zacay G, Shasha D, Bareket R, Kadim I, Hershkowitz Sikron F, Tsamir J, Mossinson D, Heymann AD. BNT162b2 vaccine effectiveness in preventing asymptomatic infection with SARS-CoV-2 virus: a nationwide historical cohort study. Open Forum Infect Dis. 2021;8(6): ofab262. https://doi.org/10.1093/ofid/ofab262 .
Martínez-Baz I, Miqueleiz A, Casado I, Navascués A, Trobajo-Sanmartín C, Burgui C, Guevara M, Ezpeleta C, Castilla J. Effectiveness of COVID-19 vaccines in preventing SARS-CoV-2 infection and hospitalisation, Navarre, Spain, January to April 2021. Eurosurveillance. 2021. https://doi.org/10.2807/1560-7917.Es.2021.26.21.2100438 .
Tenforde MW, Olson SM, Self WH, Talbot HK, Lindsell CJ, Steingrub JS, Shapiro NI, Ginde AA, Douin DJ, Prekker ME, et al. Effectiveness of Pfizer-BioNTech and moderna vaccines against COVID-19 among hospitalized adults aged ≥65 years—United States, January–March 2021. MMWR Morb Mortal Wkly Rep. 2021;70(18):674–9. https://doi.org/10.15585/mmwr.mm7018e1 .
Pawlowski C, Lenehan P, Puranik A, Agarwal V, Venkatakrishnan AJ, Niesen MJM, O’Horo JC, Virk A, Swift MD, Badley AD, et al. FDA-authorized mRNA COVID-19 vaccines are effective per real-world evidence synthesized across a multi-state health system. Med (N Y). 2021. https://doi.org/10.1016/j.medj.2021.06.007 .
Wells G, Shea B, O'Connell D, Peterson J, Welch V, Losos M, Tugwell P. The Newcastle-Ottawa Scale (NOS) for assessing the quality of nonrandomised studies in meta-analyses. http://www.ohri.ca/programs/clinical_epidemiology/oxford.asp . Accessed 20 Aug 2021.
Rostom A, Dubé C, Cranney A, et al. Celiac Disease. Rockville (MD): Agency for Healthcare Research and Quality (US); 2004 Sep. (Evidence Reports/Technology Assessments, No. 104.) Appendix D. Quality Assessment Forms. Available from: https://www.ncbi.nlm.nih.gov/books/NBK35156/ . Accessed 20 Aug 2021
Abu-Raddad LJ, Chemaitelly H, Butt AA. Effectiveness of the BNT162b2 COVID-19 vaccine against the B.1.1.7 and B.1.351 Variants. N Engl J Med. 2021;385(2):187–9. https://doi.org/10.1056/NEJMc2104974 .
Angel Y, Spitzer A, Henig O, Saiag E, Sprecher E, Padova H, Ben-Ami R. Association between vaccination with BNT162b2 and incidence of symptomatic and asymptomatic SARS-CoV-2 infections among health care workers. JAMA. 2021;325(24):2457–65. https://doi.org/10.1001/jama.2021.7152 .
Azamgarhi T, Hodgkinson M, Shah A, Skinner JA, Hauptmannova I, Briggs TWR, Warren S. BNT162b2 vaccine uptake and effectiveness in UK healthcare workers—a single centre cohort study. Nat Commun. 2021;12(1):3698. https://doi.org/10.1038/s41467-021-23927-x .
Bianchi FP, Germinario CA, Migliore G, Vimercati L, Martinelli A, Lobifaro A, Tafuri S, Stefanizzi P. BNT162b2 mRNA COVID-19 vaccine effectiveness in the prevention of SARS-CoV-2 infection: a preliminary report. J Infect Dis. 2021. https://doi.org/10.1093/infdis/jiab262 .
Britton A, Jacobs Slifka KM, Edens C, Nanduri SA, Bart SM, Shang N, Harizaj A, Armstrong J, Xu K, Ehrlich HY, et al. Effectiveness of the Pfizer-BioNTech COVID-19 vaccine among residents of two skilled nursing facilities experiencing COVID-19 outbreaks—Connecticut, December 2020–February 2021. MMWR Morb Mortal Wkly Rep. 2021;70(11):396–401. https://doi.org/10.15585/mmwr.mm7011e3 .
Cavanaugh AM, Fortier S, Lewis P, Arora V, Johnson M, George K, Tobias J, Lunn S, Miller T, Thoroughman D, et al. COVID-19 outbreak associated with a SARS-CoV-2 R1 lineage variant in a skilled nursing facility after vaccination program—Kentucky, March 2021. MMWR Morb Mortal Wkly Rep. 2021;70(17):639–43. https://doi.org/10.15585/mmwr.mm7017e2 .
Chemaitelly H, Yassine HM, Benslimane FM, Al Khatib HA, Tang P, Hasan MR, Malek JA, Coyle P, Ayoub HH, Al Kanaani Z, et al. mRNA-1273 COVID-19 vaccine effectiveness against the B.1.1.7 and B.1.351 variants and severe COVID-19 disease in Qatar. Nat Med. 2021. https://doi.org/10.1038/s41591-021-01446-y .
Chodick G, Tene L, Patalon T, Gazit S, Ben Tov A, Cohen D, Muhsen K. Assessment of effectiveness of 1 dose of BNT162b2 vaccine for SARS-CoV-2 infection 13 to 24 days after immunization. JAMA Netw Open. 2021;4(6): e2115985. https://doi.org/10.1001/jamanetworkopen.2021.15985 .
Chodick G, Tene L, Rotem RS, Patalon T, Gazit S, Ben-Tov A, Weil C, Goldshtein I, Twig G, Cohen D, et al. The effectiveness of the TWO-DOSE BNT162b2 vaccine: analysis of real-world data. Clin Infect Dis. 2021. https://doi.org/10.1093/cid/ciab438 .
Dagan N, Barda N, Kepten E, Miron O, Perchik S, Katz MA, Hernán MA, Lipsitch M, Reis B, Balicer RD. BNT162b2 mRNA COVID-19 vaccine in a nationwide mass vaccination setting. N Engl J Med. 2021;384(15):1412–23. https://doi.org/10.1056/NEJMoa2101765 .
Flacco ME, Soldato G, Acuti Martellucci C, Carota R, Di Luzio R, Caponetti A, Manzoli L. Interim estimates of COVID-19 vaccine effectiveness in a mass vaccination setting: data from an Italian Province. VacCInes (Basel). 2021. https://doi.org/10.3390/vaccines9060628 .
Haas EJ, Angulo FJ, McLaughlin JM, Anis E, Singer SR, Khan F, Brooks N, Smaja M, Mircus G, Pan K, et al. Impact and effectiveness of mRNA BNT162b2 vaccine against SARS-CoV-2 infections and COVID-19 cases, hospitalisations, and deaths following a nationwide vaccination campaign in Israel: an observational study using national surveillance data. Lancet. 2021;397(10287):1819–29. https://doi.org/10.1016/s0140-6736(21)00947-8 .
Hall VJ, Foulkes S, Saei A, Andrews N, Oguti B, Charlett A, Wellington E, Stowe J, Gillson N, Atti A, et al. COVID-19 vaccine coverage in health-care workers in England and effectiveness of BNT162b2 mRNA vaccine against infection (SIREN): a prospective, multicentre, cohort study. Lancet. 2021;397(10286):1725–35. https://doi.org/10.1016/s0140-6736(21)00790-x .
Hyams C, Marlow R, Maseko Z, King J, Ward L, Fox K, Heath R, Tuner A, Friedrich Z, Morrison L, et al. Effectiveness of BNT162b2 and ChAdOx1 nCoV-19 COVID-19 vaccination at preventing hospitalisations in people aged at least 80 years: a test-negative, case-control study. Lancet Infect Dis. 2021. https://doi.org/10.1016/s1473-3099(21)00330-3 .
Khan N, Mahmud N. Effectiveness of SARS-CoV-2 vaccination in a veterans affairs cohort of patients with inflammatory bowel disease with diverse exposure to immunosuppressive medications. Gastroenterology. 2021. https://doi.org/10.1053/j.gastro.2021.05.044 .
Knobel P, Serra C, Grau S, Ibañez R, Diaz P, Ferrández O, Villar R, Lopez AF, Pujolar N, Horcajada JP, et al. COVID-19 mRNA vaccine effectiveness in asymptomatic healthcare workers. Infect Control Hosp Epidemiol. 2021. https://doi.org/10.1017/ice.2021.287 .
Lopez Bernal J, Andrews N, Gower C, Robertson C, Stowe J, Tessier E, Simmons R, Cottrell S, Roberts R, O’Doherty M, et al. Effectiveness of the Pfizer-BioNTech and Oxford-AstraZeneca vaccines on covid-19 related symptoms, hospital admissions, and mortality in older adults in England: test negative case-control study. BMJ. 2021;373: n1088. https://doi.org/10.1136/bmj.n1088 .
Mazagatos C, Monge S, Olmedo C, Vega L, Gallego P, Martín-Merino E, Sierra MJ, Limia A, Larrauri A. Effectiveness of mRNA COVID-19 vaccines in preventing SARS-CoV-2 infections and COVID-19 hospitalisations and deaths in elderly long-term care facility residents, Spain, weeks 53, 2020 to 13 2021. Eurosurveillance. 2021. https://doi.org/10.2807/1560-7917.Es.2021.26.24.2100452 .
Pilishvili T, Fleming-Dutra KE, Farrar JL, Gierke R, Mohr NM, Talan DA, Krishnadasan A, Harland KK, Smithline HA, Hou PC, et al. Interim estimates of vaccine effectiveness of Pfizer-BioNTech and Moderna COVID-19 vaccines among health care personnel—33 US Sites, January–March 2021. MMWR Morb Mortal Wkly Rep. 2021;70(20):753–8. https://doi.org/10.15585/mmwr.mm7020e2 .
Sheikh A, McMenamin J, Taylor B, Robertson C. SARS-CoV-2 Delta VOC in Scotland: demographics, risk of hospital admission, and vaccine effectiveness. Lancet. 2021;397(10293):2461–2. https://doi.org/10.1016/s0140-6736(21)01358-1 .
Shrotri M, Krutikov M, Palmer T, Giddings R, Azmi B, Subbarao S, Fuller C, Irwin-Singer A, Davies D, Tut G, et al. Vaccine effectiveness of the first dose of ChAdOx1 nCoV-19 and BNT162b2 against SARS-CoV-2 infection in residents of long-term care facilities in England (VIVALDI): a prospective cohort study. Lancet Infect Dis. 2021. https://doi.org/10.1016/s1473-3099(21)00289-9 .
Skowronski DM, Setayeshgar S, Zou M, Prystajecky N, Tyson JR, Galanis E, Naus M, Patrick DM, Sbihi H, El Adam S, et al. Single-dose mRNA vaccine effectiveness against SARS-CoV-2, including Alpha and Gamma variants: a test-negative design in adults 70 years and older in British Columbia,Canada. Clin Infect Dis. 2021. https://doi.org/10.1093/cid/ciab616 .
Swift MD, Breeher LE, Tande AJ, Tommaso CP, Hainy CM, Chu H, Murad MH, Berbari EF, Virk A. Effectiveness of mRNA COVID-19 vaccines against SARS-CoV-2 infection in a cohort of healthcare personnel. Clin Infect Dis. 2021. https://doi.org/10.1093/cid/ciab361 .
Thompson MG, Burgess JL, Naleway AL, Tyner H, Yoon SK, Meece J, Olsho LEW, Caban-Martinez AJ, Fowlkes AL, Lutrick K, et al. Prevention and attenuation of COVID-19 with the BNT162b2 and mRNA-1273 Vaccines. N Engl J Med. 2021. https://doi.org/10.1056/NEJMoa2107058 .
Vasileiou E, Simpson CR, Shi T, Kerr S, Agrawal U, Akbari A, Bedston S, Beggs J, Bradley D, Chuter A, et al. Interim findings from first-dose mass COVID-19 vaccination roll-out and COVID-19 hospital admissions in Scotland: a national prospective cohort study. Lancet. 2021;397(10285):1646–57. https://doi.org/10.1016/s0140-6736(21)00677-2 .
Williams C, Al-Bargash D, Macalintal C, Stuart R, Seth A, Latham J, Gitterman L, Fedsin S, Godoy M, Kozak R, et al. COVID-19 outbreak associated with a SARS-CoV-2 P.1 lineage in a long-term care home after implementation of a vaccination program—Ontario, April–May 2021. Clin Infect Dis. 2021. https://doi.org/10.1093/cid/ciab617 .
Alhazmi A, Alamer E, Daws D, Hakami M, Darraj M, Abdelwahab S, Maghfuri A, Algaissi A. Evaluation of side effects associated with COVID-19 vaccines in Saudi Arabia. Vaccines (Basel). 2021. https://doi.org/10.3390/vaccines9060674 .
Andrzejczak-Grządko S, Czudy Z, Donderska M. Side effects after COVID-19 vaccinations among residents of Poland. Eur Rev Med Pharmacol Sci. 2021;25(12):4418–21. https://doi.org/10.26355/eurrev_202106_26153 .
Baldolli A, Michon J, Appia F, Galimard C, Verdon R, Parienti JJ. Tolerance of BNT162b2 mRNA COVI-19 vaccine in patients with a medical history of COVID-19 disease: a case control study. Vaccine. 2021;39(32):4410–3. https://doi.org/10.1016/j.vaccine.2021.06.054 .
Cherian S, Paul A, Ahmed S, Alias B, Manoj M, Santhosh AK, Varghese DR, Krishnan N, Shenoy P. Safety of the ChAdOx1 nCoV-19 and the BBV152 vaccines in 724 patients with rheumatic diseases: a post-vaccination cross-sectional survey. Rheumatol Int. 2021;41(8):1441–5. https://doi.org/10.1007/s00296-021-04917-0 .
Chevallier P, Coste-Burel M, Le Bourgeois A, Peterlin P, Garnier A, Béné MC, Imbert BM, Drumel T, Le Gouill S, Moreau P, et al. Safety and immunogenicity of a first dose of SARS-CoV-2 mRNA vaccine in allogeneic hematopoietic stem-cells recipients. EJHaem. 2021. https://doi.org/10.1002/jha2.242 .
Connolly CM, Ruddy JA, Boyarsky BJ, Avery RK, Werbel WA, Segev DL, Garonzik-Wang J, Paik JJ. Safety of the first dose of mRNA SARS-CoV-2 vaccines in patients with rheumatic and musculoskeletal diseases. Ann Rheum Dis. 2021. https://doi.org/10.1136/annrheumdis-2021-220231 .
Furer V, Eviatar T, Zisman D, Peleg H, Paran D, Levartovsky D, Zisapel M, Elalouf O, Kaufman I, Meidan R, et al. Immunogenicity and safety of the BNT162b2 mRNA COVID-19 vaccine in adult patients with autoimmune inflammatory rheumatic diseases and in the general population: a multicentre study. Ann Rheum Dis. 2021. https://doi.org/10.1136/annrheumdis-2021-220647 .
Gee J, Marquez P, Su J, Calvert GM, Liu R, Myers T, Nair N, Martin S, Clark T, Markowitz L, et al. First month of COVID-19 vaccine safety monitoring—United States, December 14, 2020–January 13, 2021. MMWR Morb Mortal Wkly Rep. 2021;70(8):283–8. https://doi.org/10.15585/mmwr.mm7008e3 .
Hashimoto T, Ozaki A, Bhandari D, Sawano T, Sah R, Tanimoto T. High anaphylaxis rates following vaccination with the Pfizer BNT162b2 mRNA vaccine against COVID-19 in Japanese health care workers; a secondary analysis of initial post-approval safety data. J Travel Med. 2021. https://doi.org/10.1093/jtm/taab090 .
Lv G, Yuan J, Xiong X, Li M. Mortality rate and characteristics of deaths following COVID-19 vaccination. Front Med (Lausanne). 2021;8: 670370. https://doi.org/10.3389/fmed.2021.670370 .
McMurry R, Lenehan P, Awasthi S, Silvert E, Puranik A, Pawlowski C, Venkatakrishnan AJ, Anand P, Agarwal V, O’Horo JC, et al. Real-time analysis of a mass vaccination effort confirms the safety of FDA-authorized mRNA COVID-19 vaccines. Med (N Y). 2021. https://doi.org/10.1016/j.medj.2021.06.006 .
Monin L, Laing AG, Muñoz-Ruiz M, McKenzie DR, Del Molino Del Barrio I, Alaguthurai T, Domingo-Vila C, Hayday TS, Graham C, Seow J, et al. Safety and immunogenicity of one versus two doses of the COVID-19 vaccine BNT162b2 for patients with cancer: interim analysis of a prospective observational study. Lancet Oncol. 2021;22(6):765–78. https://doi.org/10.1016/s1470-2045(21)00213-8 .
Pagotto V, Ferloni A, Mercedes Soriano M, Díaz M, Braguinsky Golde N, González MI, Asprea V, Staneloni MI, Zingoni P, Vidal G, et al. Active monitoring of early safety of Sputnik V vaccine in Buenos Aires, Argentina. MediCIna (B Aires). 2021;81(3):408–14.
Google Scholar
Peled Y, Ram E, Lavee J, Sternik L, Segev A, Wieder-Finesod A, Mandelboim M, Indenbaum V, Levy I, Raanani E, et al. BNT162b2 vaccination in heart transplant recipients: Clinical experience and antibody response. J Heart Lung Transplant. 2021. https://doi.org/10.1016/j.healun.2021.04.003 .
Quiroga B, Sánchez-Álvarez E, Goicoechea M, de Sequera P. COVID-19 vaccination among Spanish nephrologists: acceptance and side effects. J Healthc Qual Res. 2021. https://doi.org/10.1016/j.jhqr.2021.05.002 .
Ram R, Hagin D, Kikozashvilli N, Freund T, Amit O, Bar-On Y, Beyar-Katz O, Shefer G, Moshiashvili MM, Karni C, et al. Safety and immunogenicity of the BNT162b2 mRNA COVID-19 vaccine in patients after allogeneic HCT or CD19-based CART therapy—a single center prospective cohort study. Transplant Cell Ther. 2021. https://doi.org/10.1016/j.jtct.2021.06.024 .
Revon-Riviere G, Ninove L, Min V, Rome A, Coze C, Verschuur A, de Lamballerie X, André N. The BNT162b2 mRNA COVID-19 vaccine in adolescents and young adults with cancer: a monocentric experience. Eur J Cancer. 2021;154:30–4. https://doi.org/10.1016/j.ejca.2021.06.002 .
Riad A, Pokorná A, Mekhemar M, Conrad J, Klugarová J, Koščík M, Klugar M, Attia S. Safety of ChAdOx1 nCoV-19 vaccine: independent evidence from two EU states. Vaccines (Basel). 2021. https://doi.org/10.3390/vaccines9060673 .
Riad A, Sağıroğlu D, Üstün B, Pokorná A, Klugarová J, Attia S, Klugar M. Prevalence and risk factors of CoronaVac Side effects: an independent cross-sectional study among healthcare workers in Turkey. J Clin Med. 2021. https://doi.org/10.3390/jcm10122629 .
Rosman Y, Lavi N, Meir-Shafrir K, Lachover-Roth I, Cohen-Engler A, Mekori YA, Confino-Cohen R. Safety of BNT162b2 mRNA COVID-19 vaccine in patients with mast cell disorders. J Allergy Clin Immunol Pract. 2021. https://doi.org/10.1016/j.jaip.2021.06.032 .
Signorelli C, Odone A, Gianfredi V, Capraro M, Kacerik E, Chiecca G, Scardoni A, Minerva M, Mantecca R, Musarò P, et al. Application of the “immunization islands” model to improve quality, efficiency and safety of a COVID-19 mass vaccination site. Ann Ig. 2021;33(5):499–512. https://doi.org/10.7416/ai.2021.2456 .
Vallée A, Chan-Hew-Wai A, Bonan B, Lesprit P, Parquin F, Catherinot É, Choucair J, Billard D, Amiel-Taieb C, Camps È, et al. Oxford-AstraZeneca COVID-19 vaccine: need of a reasoned and effective vaccine campaign. Public Health. 2021;196:135–7. https://doi.org/10.1016/j.puhe.2021.05.030 .
Wang J, Hou Z, Liu J, Gu Y, Wu Y, Chen Z, Ji J, Diao S, Qiu Y, Zou S, et al. Safety and immunogenicity of COVID-19 vaccination in patients with non-alcoholic fatty liver disease (CHESS2101): a multicenter study. J Hepatol. 2021. https://doi.org/10.1016/j.jhep.2021.04.026 .
Zhang MX, Zhang TT, Shi GF, Cheng FM, Zheng YM, Tung TH, Chen HX. Safety of an inactivated SARS-CoV-2 vaccine among healthcare workers in China. Expert Rev Vaccines. 2021. https://doi.org/10.1080/14760584.2021.1925112 .
Shay DK, Gee J, Su JR, Myers TR, Marquez P, Liu R, Zhang B, Licata C, Clark TA, Shimabukuro TT. Safety monitoring of the Janssen (Johnson & Johnson) COVID-19 Vaccine—United States, March–April 2021. MMWR Morb Mortal Wkly Rep. 2021;70(18):680–4. https://doi.org/10.15585/mmwr.mm7018e2 .
Prevention CCfDCa. Information analysis of COVID-19 vaccine adverse reaction monitoring in China. 2021-5-28. http://www.chinacdc.cn/jkzt/ymyjz/ymyjjz_6758/202105/t20210528_230908.html . Accessed 20 Aug 2021.
Kow CS, Hasan SS. Real-world effectiveness of BNT162b2 mRNA vaccine: a meta-analysis of large observational studies. Inflammopharmacology. 2021;29(4):1075–90. https://doi.org/10.1007/s10787-021-00839-2 .
Angyal A, Longet S, Moore S, Payne RP, Harding A et al. T-Cell and Antibody Responses to First BNT162b2 Vaccine Dose in Previously SARS-CoV-2-Infected and Infection-Naive UK Healthcare Workers: A Multicentre, Prospective, Observational Cohort Study. Available at SSRN: https://ssrn.com/abstract=3820576 or https://doi.org/10.2139/ssrn.3820576 . Accessed 20 Aug 2021.
Pimenta D, Yates C, Pagel C, Gurdasani D. Delaying the second dose of covid-19 vaccines. BMJ. 2021;372: n710. https://doi.org/10.1136/bmj.n710 .
Tauh T, Mozel M, Meyler P, Lee SM. An updated look at the 16-week window between doses of vaccines in BC for COVID-19. BC Med J. 2021;63(3):102–3.
Kadire SR, Wachter RM, Lurie N. Delayed second dose versus standard regimen for COVID-19 vaccination. N Engl J Med. 2021;384(9): e28. https://doi.org/10.1056/NEJMclde2101987 .
Bergwerk M, Gonen T, Lustig Y, Amit S, Lipsitch M, Cohen C, Mandelboim M, Gal Levin E, Rubin C, Indenbaum V, et al. COVID-19 breakthrough infections in vaccinated health care workers. N Engl J Med. 2021. https://doi.org/10.1056/NEJMoa2109072 .
Truong TT, Ryutov A, Pandey U, Yee R, Goldberg L, Bhojwani D, Aguayo-Hiraldo P, Pinsky BA, Pekosz A, Shen L, et al. Persistent SARS-CoV-2 infection and increasing viral variants in children and young adults with impaired humoral immunity. medRxiv. 2021. https://doi.org/10.1101/2021.02.27.21252099 .
Choi B, Choudhary MC, Regan J, Sparks JA, Padera RF, Qiu X, Solomon IH, Kuo HH, Boucau J, Bowman K, et al. Persistence and evolution of SARS-CoV-2 in an Immunocompromised Host. N Engl J Med. 2020;383(23):2291–3. https://doi.org/10.1056/NEJMc2031364 .
Corey L, Beyrer C, Cohen MS, Michael NL, Bedford T, Rolland M. SARS-CoV-2 variants in patients with immunosuppression. N Engl J Med. 2021;385(6):562–6. https://doi.org/10.1056/NEJMsb2104756 .
Bar-On YM, Goldberg Y, Mandel M, Bodenheimer O, Freedman L, Kalkstein N, Mizrahi B, Alroy-Preis S, Ash N, Milo R, et al. Protection of BNT162b2 vaccine booster against Covid-19 in Israel. N Engl J Med. 2021;385(15):1393–400. https://doi.org/10.1056/NEJMoa2114255 .
Hause AM, Baggs J, Gee J, Marquez P, Myers TR, Shimabukuro TT, Shay DK. Safety monitoring of an additional dose of COVID-19 vaccine—United States, August 12–September 19, 2021. MMWR Morb Mortal Wkly Rep. 2021;70(39):1379–84. https://doi.org/10.15585/mmwr.mm7039e4 .
Furlow B. Immunocompromised patients in the USA and UK should receive third dose of COVID-19 vaccine. Lancet Rheumatol. 2021. https://doi.org/10.1016/s2665-9913(21)00313-1 .
Flaxman A, Marchevsky NG, Jenkin D, Aboagye J, Aley PK, Angus B, Belij-Rammerstorfer S, Bibi S, Bittaye M, Cappuccini F, et al. Reactogenicity and immunogenicity after a late second dose or a third dose of ChAdOx1 nCoV-19 in the UK: a substudy of two randomised controlled trials (COV001 and COV002). Lancet. 2021;398(10304):981–90. https://doi.org/10.1016/s0140-6736(21)01699-8 .
Peled Y, Ram E, Lavee J, Segev A, Matezki S, Wieder-Finesod A, Halperin R, Mandelboim M, Indenbaum V, Levy I, et al. Third dose of the BNT162b2 vaccine in heart transplant recipients: immunogenicity and clinical experience. J Heart Lung Transplant. 2021. https://doi.org/10.1016/j.healun.2021.08.010 .
WHO. WHO press conference on coronavirus disease (COVID-19)—4 August 2021. 2021. https://www.who.int/multi-media/details/who-press-conference-on-coronavirus-disease-(covid-19)---4-august-2021 . Accessed 20 Aug 2021.
Cascella M, Rajnik M, Aleem A, Dulebohn SC, Di Napoli R. Features, evaluation, and treatment of coronavirus (COVID-19). In: StatPearls. edn. Treasure Island (FL): StatPearls Publishing Copyright © 2021, StatPearls Publishing LLC.; 2021.
Goshen-Lago T, Waldhorn I, Holland R, Szwarcwort-Cohen M, Reiner-Benaim A, Shachor-Meyouhas Y, Hussein K, Fahoum L, Baruch M, Peer A, et al. Serologic status and toxic effects of the SARS-CoV-2 BNT162b2 vaccine in patients undergoing treatment for cancer. JAMA Oncol. 2021. https://doi.org/10.1001/jamaoncol.2021.2675 .
Ou MT, Boyarsky BJ, Motter JD, Greenberg RS, Teles AT, Ruddy JA, Krach MR, Jain VS, Werbel WA, Avery RK, et al. Safety and reactogenicity of 2 doses of SARS-CoV-2 vaccination in solid organ transplant recipients. Transplantation. 2021. https://doi.org/10.1097/tp.0000000000003780 .
Bookstein Peretz S, Regev N, Novick L, Nachshol M, Goffer E, Ben-David A, Asraf K, Doolman R, Sapir E, Regev Yochay G, et al. Short-term outcome of pregnant women vaccinated by BNT162b2 mRNA COVID-19 vaccine. Ultrasound Obstet Gynecol. 2021. https://doi.org/10.1002/uog.23729 .
Shimabukuro TT, Kim SY, Myers TR, Moro PL, Oduyebo T, Panagiotakopoulos L, Marquez PL, Olson CK, Liu R, Chang KT, et al. Preliminary findings of mRNA COVID-19 vaccine safety in pregnant persons. N Engl J Med. 2021;384(24):2273–82. https://doi.org/10.1056/NEJMoa2104983 .
Peled Y, Ram E, Lavee J, Sternik L, Segev A, Wieder-Finesod A, Mandelboim M, Indenbaum V, Levy I, Raanani E, et al. BNT162b2 vaccination in heart transplant recipients: clinical experience and antibody response. J Heart Lung Transplant. 2021;40(8):759–62. https://doi.org/10.1016/j.healun.2021.04.003 .
Thomas SJ, Moreira ED Jr, Kitchin N, Absalon J, Gurtman A, Lockhart S, Perez JL, Pérez Marc G, Polack FP, Zerbini C, et al. Safety and efficacy of the BNT162b2 mRNA COVID-19 vaccine through 6 months. N Engl J Med. 2021. https://doi.org/10.1056/NEJMoa2110345 .
Falsey AR, Sobieszczyk ME, Hirsch I, Sproule S, Robb ML, Corey L, Neuzil KM, Hahn W, Hunt J, Mulligan MJ, et al. Phase 3 safety and efficacy of AZD1222 (ChAdOx1 nCoV-19) COVID-19 vaccine. N Engl J Med. 2021. https://doi.org/10.1056/NEJMoa2105290 .
El Sahly HM, Baden LR, Essink B, Doblecki-Lewis S, Martin JM, Anderson EJ, Campbell TB, Clark J, Jackson LA, Fichtenbaum CJ, et al. Efficacy of the mRNA-1273 SARS-CoV-2 vaccine at completion of blinded phase. N Engl J Med. 2021. https://doi.org/10.1056/NEJMoa2113017 .
Tanriover MD, Doğanay HL, Akova M, Güner HR, Azap A, Akhan S, Köse Ş, Erdinç F, Akalın EH, Tabak ÖF, et al. Efficacy and safety of an inactivated whole-virion SARS-CoV-2 vaccine (CoronaVac): interim results of a double-blind, randomised, placebo-controlled, phase 3 trial in Turkey. Lancet. 2021;398(10296):213–22. https://doi.org/10.1016/s0140-6736(21)01429-x .
Kumar S, Saurabh MK, Maharshi V. Efficacy and safety of potential vaccine candidates against coronavirus disease 2019: a systematic review. J Adv Pharm Technol Res. 2021;12(3):215–21. https://doi.org/10.4103/japtr.JAPTR_229_20 .
Burgos-Salcedo J. A rational strategy to support approved COVID-19 vaccines prioritization. Hum Vaccin Immunother. 2021;17(10):3474–7. https://doi.org/10.1080/21645515.2021.1922060 .
Download references
Acknowledgements
This study was funded by the National Natural Science Foundation of China (72122001; 71934002) and the National Science and Technology Key Projects on Prevention and Treatment of Major infectious disease of China (2020ZX10001002). The funders had no role in study design, data collection and analysis, decision to publish, or preparation of the paper. No payment was received by any of the co-authors for the preparation of this article.
Author information
Qiao Liu and Chenyuan Qin are joint first authors
Authors and Affiliations
Department of Epidemiology and Biostatistics, School of Public Health, Peking University, Beijing, 100191, China
Qiao Liu, Chenyuan Qin, Min Liu & Jue Liu
Institute for Global Health and Development, Peking University, Beijing, 100871, China
Chenyuan Qin & Jue Liu
You can also search for this author in PubMed Google Scholar
Contributions
LQ and QCY contributed equally as first authors. LJ and LM contributed equally as correspondence authors. LJ and LM conceived and designed the study; LQ, QCY and LJ carried out the literature searches, extracted the data, and assessed the study quality; LQ and QCY performed the statistical analysis and wrote the manuscript; LJ, LM, LQ and QCY revised the manuscript. All authors read and approved the final manuscript.
Corresponding authors
Correspondence to Min Liu or Jue Liu .
Ethics declarations
Ethics approval and consent to participate.
Not applicable.
Consent for publication
Competing interests.
The authors have no conflicts of interest to declare that are relevant to the content of this article.
Supplementary Information
Additional file 1: table s1..
Characteristic of studies included for vaccine effectiveness.
Additional file 2: Table S2.
Characteristic of studies included for vaccine safety.
Rights and permissions
Open Access This article is licensed under a Creative Commons Attribution 4.0 International License, which permits use, sharing, adaptation, distribution and reproduction in any medium or format, as long as you give appropriate credit to the original author(s) and the source, provide a link to the Creative Commons licence, and indicate if changes were made. The images or other third party material in this article are included in the article's Creative Commons licence, unless indicated otherwise in a credit line to the material. If material is not included in the article's Creative Commons licence and your intended use is not permitted by statutory regulation or exceeds the permitted use, you will need to obtain permission directly from the copyright holder. To view a copy of this licence, visit http://creativecommons.org/licenses/by/4.0/ . The Creative Commons Public Domain Dedication waiver ( http://creativecommons.org/publicdomain/zero/1.0/ ) applies to the data made available in this article, unless otherwise stated in a credit line to the data.
Reprints and permissions
About this article
Cite this article.
Liu, Q., Qin, C., Liu, M. et al. Effectiveness and safety of SARS-CoV-2 vaccine in real-world studies: a systematic review and meta-analysis. Infect Dis Poverty 10 , 132 (2021). https://doi.org/10.1186/s40249-021-00915-3
Download citation
Received : 07 September 2021
Accepted : 01 November 2021
Published : 14 November 2021
DOI : https://doi.org/10.1186/s40249-021-00915-3
Share this article
Anyone you share the following link with will be able to read this content:
Sorry, a shareable link is not currently available for this article.
Provided by the Springer Nature SharedIt content-sharing initiative
- Effectiveness
- Meta-analysis
Infectious Diseases of Poverty
ISSN: 2049-9957
- Submission enquiries: Access here and click Contact Us
- General enquiries: [email protected]
- Our Program Divisions
- Our Three Academies
- Government Affairs
- Statement on Diversity and Inclusion
- Our Study Process
- Conflict of Interest Policies and Procedures
- Project Comments and Information
- Read Our Expert Reports and Published Proceedings
- Explore PNAS, the Flagship Scientific Journal of NAS
- Access Transportation Research Board Publications
- Coronavirus Disease 2019 (COVID-19)
- Diversity, Equity, and Inclusion
- Economic Recovery
- Fellowships and Grants
- Publications by Division
- Division of Behavioral and Social Sciences and Education
- Division on Earth and Life Studies
- Division on Engineering and Physical Sciences
- Gulf Research Program
- Health and Medicine Division
- Policy and Global Affairs Division
- Transportation Research Board
- National Academy of Sciences
- National Academy of Engineering
- National Academy of Medicine
- Publications by Topic
- Agriculture
- Behavioral and Social Sciences
- Biography and Autobiography
- Biology and Life Sciences
- Computers and Information Technology
- Conflict and Security Issues
- Earth Sciences
- Energy and Energy Conservation
- Engineering and Technology
- Environment and Environmental Studies
- Food and Nutrition
- Health and Medicine
- Industry and Labor
- Math, Chemistry, and Physics
- Policy for Science and Technology
- Space and Aeronautics
- Surveys and Statistics
- Transportation and Infrastructure
- Searchable Collections
- New Releases
VIEW LARGER COVER
Evidence Review of the Adverse Effects of COVID-19 Vaccination and Intramuscular Vaccine Administration
Vaccines are a public health success story, as they have prevented or lessened the effects of many infectious diseases. To address concerns around potential vaccine injuries, the Health Resources and Services Administration (HRSA) administers the Vaccine Injury Compensation Program (VICP) and the Countermeasures Injury Compensation Program (CICP), which provide compensation to those who assert that they were injured by routine vaccines or medical countermeasures, respectively. The National Academies of Sciences, Engineering, and Medicine have contributed to the scientific basis for VICP compensation decisions for decades.
HRSA asked the National Academies to convene an expert committee to review the epidemiological, clinical, and biological evidence about the relationship between COVID-19 vaccines and specific adverse events, as well as intramuscular administration of vaccines and shoulder injuries. This report outlines the committee findings and conclusions.
RESOURCES AT A GLANCE
- Press Release
- Digital Resource: Evidence Review of the Adverse Effects of COVID-19 Vaccination
- Digital Resource: Evidence Review of Shoulder Injuries from Intramuscular Administration of Vaccines
- Health and Medicine — Health Sciences
- Health and Medicine — Public Health and Prevention
- Health and Medicine — Policy, Reviews and Evaluations
Suggested Citation
National Academies of Sciences, Engineering, and Medicine. 2024. Evidence Review of the Adverse Effects of COVID-19 Vaccination and Intramuscular Vaccine Administration . Washington, DC: The National Academies Press. https://doi.org/10.17226/27746. Import this citation to: Bibtex EndNote Reference Manager
Publication Info
- Prepublication: 978-0-309-71829-5
- Paperback (forthcoming): 978-0-309-71832-5
What is skim?
The Chapter Skim search tool presents what we've algorithmically identified as the most significant single chunk of text within every page in the chapter. You may select key terms to highlight them within pages of each chapter.
Copyright Information
The National Academies Press (NAP) has partnered with Copyright Clearance Center's Marketplace service to offer you a variety of options for reusing NAP content. Through Marketplace, you may request permission to reprint NAP content in another publication, course pack, secure website, or other media. Marketplace allows you to instantly obtain permission, pay related fees, and print a license directly from the NAP website. The complete terms and conditions of your reuse license can be found in the license agreement that will be made available to you during the online order process. To request permission through Marketplace you are required to create an account by filling out a simple online form. The following list describes license reuses offered by the NAP through Marketplace:
- Republish text, tables, figures, or images in print
- Post on a secure Intranet/Extranet website
- Use in a PowerPoint Presentation
- Distribute via CD-ROM
Click here to obtain permission for the above reuses. If you have questions or comments concerning the Marketplace service, please contact:
Marketplace Support International +1.978.646.2600 US Toll Free +1.855.239.3415 E-mail: [email protected] marketplace.copyright.com
To request permission to distribute a PDF, please contact our Customer Service Department at [email protected] .
What is a prepublication?

An uncorrected copy, or prepublication, is an uncorrected proof of the book. We publish prepublications to facilitate timely access to the committee's findings.
You can purchase the uncorrected copy now, or pre-order the final version of the book. Prepublication sales are final. Due to the nature of this type of publication, it is not returnable.
What happens when I pre-order?
The final version of this book has not been published yet. You can pre-order a copy of the book and we will send it to you when it becomes available. We will not charge you for the book until it ships. Pricing for a pre-ordered book is estimated and subject to change. All backorders will be released at the final established price. As a courtesy, if the price increases by more than $3.00 we will notify you. If the price decreases, we will simply charge the lower price. Applicable discounts will be extended.
Downloading and Using eBooks from NAP
What is an ebook.
An ebook is one of two file formats that are intended to be used with e-reader devices and apps such as Amazon Kindle or Apple iBooks.
Why is an eBook better than a PDF?
A PDF is a digital representation of the print book, so while it can be loaded into most e-reader programs, it doesn't allow for resizable text or advanced, interactive functionality. The eBook is optimized for e-reader devices and apps, which means that it offers a much better digital reading experience than a PDF, including resizable text and interactive features (when available).
Where do I get eBook files?
eBook files are now available for a large number of reports on the NAP.edu website. If an eBook is available, you'll see the option to purchase it on the book page.
View more FAQ's about Ebooks
Types of Publications
Consensus Study Report: Consensus Study Reports published by the National Academies of Sciences, Engineering, and Medicine document the evidence-based consensus on the study’s statement of task by an authoring committee of experts. Reports typically include findings, conclusions, and recommendations based on information gathered by the committee and the committee’s deliberations. Each report has been subjected to a rigorous and independent peer-review process and it represents the position of the National Academies on the statement of task.
Serious adverse events of special interest following mRNA COVID-19 vaccination in randomized trials in adults
Affiliations.
- 1 Thibodaux Regional Health System, Thibodaux, LA, USA. Electronic address: [email protected].
- 2 Unit of Innovation and Organization, Navarre Health Service, Spain. Electronic address: [email protected].
- 3 Institute of Evidence-Based Healthcare, Bond University, Gold Coast, QLD, Australia. Electronic address: [email protected].
- 4 Fielding School of Public Health and College of Letters and Science, University of California, Los Angeles, CA, USA. Electronic address: [email protected].
- 5 Geffen School of Medicine, University of California, Los Angeles, CA, USA. Electronic address: [email protected].
- 6 Clinical Excellence Research Center, School of Medicine, Stanford University, CA, USA. Electronic address: [email protected].
- 7 School of Pharmacy, University of Maryland, Baltimore, MD, USA. Electronic address: [email protected].
- PMID: 36055877
- PMCID: PMC9428332
- DOI: 10.1016/j.vaccine.2022.08.036
Introduction: In 2020, prior to COVID-19 vaccine rollout, the Brighton Collaboration created a priority list, endorsed by the World Health Organization, of potential adverse events relevant to COVID-19 vaccines. We adapted the Brighton Collaboration list to evaluate serious adverse events of special interest observed in mRNA COVID-19 vaccine trials.
Methods: Secondary analysis of serious adverse events reported in the placebo-controlled, phase III randomized clinical trials of Pfizer and Moderna mRNA COVID-19 vaccines in adults ( NCT04368728 and NCT04470427 ), focusing analysis on Brighton Collaboration adverse events of special interest.
Results: Pfizer and Moderna mRNA COVID-19 vaccines were associated with an excess risk of serious adverse events of special interest of 10.1 and 15.1 per 10,000 vaccinated over placebo baselines of 17.6 and 42.2 (95 % CI -0.4 to 20.6 and -3.6 to 33.8), respectively. Combined, the mRNA vaccines were associated with an excess risk of serious adverse events of special interest of 12.5 per 10,000 vaccinated (95 % CI 2.1 to 22.9); risk ratio 1.43 (95 % CI 1.07 to 1.92). The Pfizer trial exhibited a 36 % higher risk of serious adverse events in the vaccine group; risk difference 18.0 per 10,000 vaccinated (95 % CI 1.2 to 34.9); risk ratio 1.36 (95 % CI 1.02 to 1.83). The Moderna trial exhibited a 6 % higher risk of serious adverse events in the vaccine group: risk difference 7.1 per 10,000 (95 % CI -23.2 to 37.4); risk ratio 1.06 (95 % CI 0.84 to 1.33). Combined, there was a 16 % higher risk of serious adverse events in mRNA vaccine recipients: risk difference 13.2 (95 % CI -3.2 to 29.6); risk ratio 1.16 (95 % CI 0.97 to 1.39).
Discussion: The excess risk of serious adverse events found in our study points to the need for formal harm-benefit analyses, particularly those that are stratified according to risk of serious COVID-19 outcomes. These analyses will require public release of participant level datasets.
Keywords: Adverse events of special interest; Brighton Collaboration; COVID-19; COVID-19 vaccines; Coalition for Epidemic Preparedness Innovations; Moderna COVID-19 vaccine mRNA-1273; NCT04368728 ; NCT04470427 ; Pfizer-BioNTech COVID-19 vaccine BNT162b2; SARS-CoV-2; Safety Platform for Emergency vACcines; Serious adverse events; Vaccines; mRNA vaccines.
Copyright © 2022 Elsevier Ltd. All rights reserved.
Publication types
- Clinical Trial, Phase III
- Randomized Controlled Trial
- COVID-19 Vaccines / adverse effects
- COVID-19* / prevention & control
- RNA, Messenger
- Randomized Controlled Trials as Topic
- Vaccination / adverse effects
- Vaccines, Synthetic
- mRNA Vaccines
- COVID-19 Vaccines
Associated data
- ClinicalTrials.gov/NCT04368728
- ClinicalTrials.gov/NCT04470427
- Research article
- Open access
- Published: 17 November 2021
The “anti-vax” movement: a quantitative report on vaccine beliefs and knowledge across social media
- Staci L Benoit ORCID: orcid.org/0000-0003-3437-2358 1 &
- Rachel F. Mauldin 1
BMC Public Health volume 21 , Article number: 2106 ( 2021 ) Cite this article
125k Accesses
33 Citations
73 Altmetric
Metrics details
Social media use has become a mainstay of communication and with that comes the exchange of factual and non-factual information. Social media has given many people the opportunity to speak their opinions without repercussions and create coalitionS of like-minded people. This also has led to the development of a community know as anti-vaxxers or vaccine deniers. This research explores the extent to which vaccine knowledge has reached on social media.
This cross sectional research explored the relationship between the spread of information regarding vaccines in relation to social media use. A sample of 2515 people over the age of 18 around the world completed the survey via a link distributed on Twitter, Facebook and Instagram. A series of questions on vaccine knowledge and beliefs were compounded to create an individual’s “knowledge score” and a “belief score”. Knowledge scores were ranked from low knowledge to high knowledge with increasing scores. Belief scores were ranked from belief in myths to disbelief in myths with higher scores. This score was then analysed, using a Welch test and post hoc testing when applicable, across demographics and questions relating to social media use.
Significant relations were found in both the knowledge and belief categories, many of which were similar findings between the two. North Americans had significantly lower knowledge and belief scores compared to all other continents. While the majority of people primarily use Facebook, Twitter users were significantly more knowledgeable. It was also found that higher education was correlated with higher knowledge and belief scores.
Conclusions
Overall, these correlations are important in determining ways to intervene into the anti-vax movement through the use of social media. Cross demographics were not analysed in this study but could be in future studies. To better understand the social media exposures related to vaccine information a follow up structured interview research study would be beneficial. Note that due to the cross sectional nature of this study, causal relationships could not be made.
Peer Review reports
Fear of vaccines dates as far back as vaccines themselves as evident by Edmund Massey's [ 29 ] Sermon titled “A sermon against the dangerous and sinful practice of inoculation.” This appears to be the first objection to any forms of inoculation to prevent disease with Massey stating “Let us not sinfully endeavour to alter the Course of Nature” [ 29 ]. Next in notable vaccine objections was when the smallpox vaccine was introduced, “many skeptics […] found it counterintuitive that deliberately infecting a person with a disease” [ 38 ]. This is when the world began to see a group of people who not only refused vaccination but made an effort to inform others of the “dangers” through propaganda. However this propaganda largely consisted of arguments of infringement of rights and anti-socialism [ 15 ].
Since the infamous 1998 paper by Andrew Wakefield, this was later retracted because it incorrectly related the MMR vaccine to autism, a group of people known as vaccine deniers or more commonly known as anti-vaxxers have been exponentially growing. A vaccine denier or anti-vaxxer will be defined in this study as someone who believes vaccines do not work, are not safe or refuse vaccines for themselves and their children if applicable. Claims about vaccine safety, efficacy, and adverse effects have been evolving and have now spread to almost every vaccine available. Surveys from the American Academy of Pediatrics found that the rate of parents who refused one or more recommended vaccines increased from 9.1% in 2006 to a staggering 16.7% in 2013 [ 30 ]. The problem being faced today is the wealth of information that is not only accessible but easily spread across social media platforms regardless of veracity. It is clear that the internet is now patching a significant time in health literacy and decision making. A survey by Fox [ 18 ] found "(72%) [Of] adult internet users say they have searched online for information about a range of health issues[...] (26%) adult internet users say they have read or watched someone else’s health experience about health or medical issues in the past 12 months. And 16% of adult internet users in the U.S. have gone online in the past 12 months to find others who share the same health concerns. There are an estimated 58 milion followers on anti-vaccination pages across socialmedia ] 1 ]. This study uses Social media propagation, to reach the study population.
The purpose of this study is to evaluate the current knowledge and beliefs about vaccines in people who use social media and the differences between scores and demographics. Past research has found that the strongest influence for positive vaccine views is having factual knowledge [ 11 ]. This study hopes to determine how much factual information is known and what differentiates social media users who have adequate and inadequate knowledge. This cross sectional study hypothesizes that there are correlations between each of the individual demographic questions and the respondent’s knowledge of vaccines. Knowing what differentiates people who have adequate and inadequate knowledge can be beneficial for determining how to reach people in future vaccine campaigns. This information could also be used in an attempt to better educate or correct misinformation by means of social media in those who classify under this study as having insufficient knowledge. Additionally this research will investigate the individual’s beliefs about vaccinations in people who use social media, whether positive or negative. Uncovering individual characteristics in the respondents with their different beliefs can be helpful for deciding how to correctly reach each person group in the future in regards to vaccinations. We can use this information for educational purposes through social media to help those who were found to have negative or lower beliefs toward vaccinations.
Knowledge about vaccines, both true and false, can be easily accessible but also easily confused. The internet has become a huge influence on vaccine knowledge [ 26 ] and the emergence of social media has created a vast community that allows multi-person discussion to happen instantaneously and with little supervision [ 9 ]. “Anti-vaccination activists use [social media] to disseminate messages, facts and beliefs that oppose some or all recommended vaccinations” [ 6 ]. Brunson [ 8 ] found that the most significant factor influencing parents online is the percent of parents within a parents online network that are nonconforming. Because of these factors refusal to vaccinate is becoming a concern of public safety.
Regardless of vaccination rates, legitimate information about vaccine safety is “not reaching parents in an effective or convincing manner” [ 19 ]. This study seeks to assess whether factual knowledge is reaching social media users. This is because it has been found that the strongest predictor of positive attitudes towards vaccines is better knowledge [ 11 ]. In a study of Serbian University students it was found that 47.9% of participants thought that giving multiple vaccines at one time overloads the immune system [ 11 ]. Further research will determine if views such as the one above are perceived across all social media users and not just students.
Investigations of the dissemination of vaccine knowledge across social media have been substantially less studied until recent years, especially with the development of the COVID-19 vaccine. In a study of vaccine attitudes in Twitter users Mitra et al. found that anti-vaccination tweets had a wider reach (seen by more people) than pro-vaccine tweets [ 31 ]. In a study of HPV vaccine tweets it was found that there was “an association between prior exposure to negative tweets about HPV vaccines and the subsequent posting of negative tweets about HPV vaccines” which allowed for the sharing of negative opinions to more susceptible people [ 14 ]. From the information aforementioned it can be predicted that the majority of information about vaccines on social media has a negative connotation. What is still unclear is the ability of the general population to distinguish between fact and fiction, and whether this information has an influence on their knowledge and eventually decisions.
To determine what questions would be used and what is to be expected by demographic results this study looked at Duggan and Brenner's [ 13 ] journal article on social media users. This study found several useful social media user characteristics. Firstly, women are statistically significantly more likely to be social media users compared to men. This study also shows that with age, there is a statistically significant progressive decline in social media use. In terms of education they found that social media use was approximately equal across all levels of education. Equity across races was also seen. This demographic data is important for determining what questions should be asked, but also what can be expected in the results.
It is important to determine what factors influence people to not vaccinate. Yaqub et al. found that “‘distrust of doctors’, ‘distrust of government sources’, and ‘distrust of pharmaceutical companies’ as reason for hesitancy” [ 40 ]. In that same study they found that very few people reported that they did not have access to adequate information. While this is important, it does not elaborate on the quality of information these people are using. A cross sectional study in Australia in 2012 found that although 92% of 452 parents reported that their children were adequately vaccinated, 52% reported concerns including but not limited to vaccine safety and source of vaccine knowledge [ 10 ]. However due to discrepancies in defining anti-vaxxers or people who are vaccine hesitant, this study will focus on what knowledge people have and not ask their vaccine practices. Rather, it will look into how far the anti-vax messages have reached and the characteristics of those participants that have or have not been swayed by these messages.
Since the collection of the data related to this research the world has faced the COVID-19 pandemic. Social media and vaccine hesitancy has become a huge topic of discussion and research relating to combating COVID-19. Several studies worldwide have indicated that older individuals, females, those with higher incomes and those with higher education levels were more likely to accept a vaccine [ 34 ]. Research in this area has exploded due to fast paced development and deployment of the COVID 19 vaccine. Globally there has been varying intention to get the COVID-19 vaccine ranging from 41 and 89% [ 17 ]. A survey conducted in the United States of America showed only 57.6% of respondents intended to be vaccinated but also that “(62%) [of respondents] believed that sociopolitical factors and pressures may lead to a rushed approval for the COVID vaccine without the assurances of safety and efficacy” [ 27 ].
This research was conducted by a cross sectional multiple choice study created via Survey Monkey. Survey Monkey subscription was provided by Saint James School of Medicine and was chosen based on the platforms ability to export data to IBM's SPSS. Data of both qualitative (demographic) and quantitative (Belief and Knowledge Scores as described below) nature were collected. Survey was designed to be completed in 5–10 min. It was designed with no open ended questions, no intended question bias, and no implied judgement. Survey questions were validated by pilot participation and follow up to determine if there was any ambiguity that needed to be addressed. Demographic questions were designed to be all inclusive. Belief and Knowledge questions were based off of key arguments of vaccine deniers as determined by popular social media posts. Research of key argument involved exploring multiple social media platforms and investigating posts/comments regarding vaccine denial.
Inclusion/exclusion
Subjects were included based on completion of all parts of the survey. All demographics (country, race, gender, socioeconomic status, preferred social media platform) were included except for those under 18 years of age. Those participants under 18 were excluded because in most countries the age at which an individual can consent for medical treatment such as vaccination is 18 year old [ 39 ]. Therefore, vaccine decision making if deferred to parents and guardians over 18 years of age. Those participants who no do not consent to their information being used for research purposes (first question of the survey) were excluded from the study. Survey’s that contained any missing data/questions were excluding from analysis as scoring could not be completed. The survey was designed to not allow advancement onto next question without answering the current question. If participants clicked out of browser before completing final question their survey was invalidated and subsequently not extrapolated for analysis. Survey was available for completion from August 15, 2018 till November 1, 2018.
Snowball sampling of social media users was used. Snowball sampling was used to help perpetuate the survey through social media, where social media is the quality of referral. The study population was aimed at being as demographically diverse as possible among people who use social media. Snowball sampling was chosen in particular for its ability to perpetuate hard to reach communities, such as those who identify as vaccine deniers [ 23 ]. Additionally, using Facebook with snowball sampling is effective at diversifying the geographical scope and increasing the overall response rate [ 3 ].
Recruitment
Subjects were recruited through the three largest social media platforms (Facebook, Twitter, Instagram) via a shareable web link and asked to consent before completion of the research survey. Initial survey link was posted publicly on social media platforms outlined above with information on the survey and encouragement to further share the survey once completed. A web survey was chosen due to its ease, speed, cost, and ability to obtain a geographically diverse population [ 20 ].
All questions in the survey were only available in English language and can be found in the supplementary material, document 1 titled Survey. The first half of the survey consisted of demographics and questions pertaining to use of social media and its relation to vaccine information. The latter half of the survey had six questions relating to vaccine knowledge and six questions relating to vaccine myths. The method of scoring was designed by the authors to create a numerical scale for comparative analytics. Lack of knowledge and belief in myths is not a negative feature but more so an area of improvement and discussion. As such, the design only uses positive numerals for scoring each question. This scoring system was created by the authors of this study specifically for this research. Question content was selected by authors through observation of social media posts pertaining to vaccines (both pro vaccine and anti-vaccine content) for common misconceptions and rebuttals.
The six vaccine knowledge questions were scored on a two point scale. Questions were scored by awarding two points for the answer of belief in the vaccine statement, one point for uncertainty, and zero points for the answer of disbelief in the statement. All questions were then totaled for a score on a 12 point scale. Higher values (9–12) suggesting adequate vaccine knowledge, middle range (5–8) suggesting some vaccine knowledge but with uncertainty and lower values (0–4) suggesting inadequate vaccine knowledge. This score could then be appropriately analyzed.
The six vaccine belief questions were scored on a two point scale. Two points were given for the answer choice “disbelief in the vaccine statement”, one point for uncertainty, and zero points for the answer of belief in the statement. All questions were then totaled for a score on a 12-point scale. Similar to knowledge values, higher values were indicative of disbelief in common myths, whereas lower values indicated a belief in common myths. This score could then be appropriately analyzed.
All data analysis was conducted using IBM’s SPSS. Significance testing was performed using the Welch test. This test was chosen based on the negatively skewed data distribution with non-homogeneity of variances and sample sizes [ 16 ]. The Welch test has historically been shown to better control Type 1 error for these parameters compared to other tests [ 35 ]. Post hoc analysis was completed with Games Howell due to its robustness and utility in non-normal distributions [ 21 ]. A standard P value of 0.05 was used for statistical significance but reported up to < 0.001 which is the limit on statistical software. Raw and descriptive data is available from openICPSR.org project ID openicpsr-120,505 [ 5 ].
Of the 2517 respondents, 2417 were included in the analysis based on the inclusion/exclusion criteria. The age of participants showed 446 (18.5%) people aged 18–24, 715 (29.6%) people aged 25–34, 591 (24.5%) people aged 35–44, 394 (16.3%) people aged 45–54, 189 (7.8%) people aged 55–64 and 82 (3.4%) people over the age of 65. Females accounted for 80.1% of the respondents ( n = 1937 people) and males accounted for 18.8% ( n = 454 people). Respondents were predominantly North American with 70.3% ( n = 1700) from the USA and 12.9% ( n = 312) from other North American Countries. The remaining were divided into 7.4% ( n = 180) Australia/Oceanic, 7.3% ( n = 176) European, 0.9% ( n = 22) Asian, 0.6% ( n = 15) African, 0.5% ( n = 11) South American, and 1 respondent from Antarctica. The education levels showed that 0.1% ( n = 2) had no formal schooling, 0.4% ( n = 10) completed elementary school (grade level 1–8), 21.2% ( n = 509) completed high school (grade level 9–12/13), 22.3% ( n = 540) completed an Associates (2 year) degree, 34.5% ( n = 833) completed a Bachelor (4 year) degree, 13.9% ( n = 336) completed a Master’s degree, and 7.7% ( n = 187) completed a Professional degree (PhD, MD, DC, DO, etc.). Individuals identified themselves as Lower class socioeconomic status (SES) comprised 9.8% ( n = 238) of the population, 82.2% ( n = 1987) as Middle class SES, and 7.9% ( n = 192) as Upper class SES.
Facebook is the most commonly used social media type in the population with 69.8% ( n = 1688), followed by Twitter 15.6% ( n = 378), Instagram 12.9% ( n = 311), then other forms of social media 1.7% ( n = 40). Other forms of social media were identified as Snapchat, Tumblr, Reddit, Pinterest, or using all platforms equally. Most people 47.9% ( n = 1158) claimed to only spend 0–2 h on social media daily, followed by 40.9% ( n = 989) using 3–4 h, 8.4% ( n = 204) using 5–6 h, 1.5% ( n = 36) using 7–8 h, and 1.2% ( n = 30) using social media for over 9 h. Most respondents 92.7% ( n = 2240) have seen posts on social media about vaccines and only 7.3% ( n = 177) have not. These posts influence 5.4% ( n = 130) of users to think vaccines are worse than previously thought, 13.6% ( n = 328) to think vaccines are better than previous thought, 76.4% ( n = 1846) claim to not have been influenced by the posts, and 4.7% ( n = 113) had not seen any posts. Lastly, people claimed to trust doctors 89.4% ( n = 2160) the most with their immunization related information/decisions. The remaining people trust the internet 4.1% ( n = 100), family 2.0% ( n = 48), peers and friends 2.3% ( n = 55), social media 0.2% ( n = 5) and the government 2.0% ( n = 49) with their information and decisions.
Table 1, found in the supplementary document 2 labeled “Tables”, depicts the frequency of knowledge scores in the sample population. As described in the methods, knowledge scores are based on a scale from 0 to 12 derived from 6 questions with answers ranked from 0 to 2 points. Scores toward 0 represent negatively skewed knowledge, or lack of correct information. Scores toward 12 represent positively skewed knowledge, or adequate vaccine knowledge. Scores of 6 represent uncertainness.
Analysis of all demographic questions against the respondent’s knowledge score was completed by Welch and then further analyzed by Games Howell. Explanations of why these tests were chosen can be found in Methods. When age was compared with knowledge scores a Welch statistical value of 0.763 and the significance of 0.576 ( p > 0.05). Post hoc was not necessary.
Gender analysis showed a Welch statistic of 1.627 with a significance value of 0.204 ( p > 0.05). Post hoc analysis was not examined because there was no significance.
Geographical Welch testing showed a statistic value of 11.552 with a significance of < 0.001( p < 0.05). Since this value is statically significant post hoc analysis was examined. North Americans (USA) has significantly lower knowledge scores compared to Europe (mean difference − 0.78309, significance < 0.001), and Australia/Oceania (means difference − 0.84316, significance < 0.001). North Americans (Other) also showed significantly lower knowledge scores compared to Europe (means difference − 0.76122, significance 0.001) and Australia/Oceania (means difference − 0.84316, significance 0.001). Values from Asia, Africa, and South American should be looked at with caution because of low responses. Antarctica was excluded from these calculations because there was only one respondent.
Analysis of respondents highest level of education completed showed a Welch statistic of 13.030 and significance of 0.001 ( p < 0.05). Post hoc showed that those who completed a Professional degree had significantly higher scores than Bachelor’s degree (means difference 0.55353, significance of 0.007), Associates degree (means difference 1.21578, significance of < 0.001), and high school (means difference 1.11273, significance < 0.001). Those with Masters Degrees were significantly higher scoring than Associate degrees (means difference of 0.80000, significance < 0.001), and high school (means difference of 0.69695, significance of 0.001). Bachelor’s degree holders had significantly higher scores compared to Associates degree (means difference of 0.66224, significance of < 0.001) and High school (means difference of 0.55920, significance of 0.003). Those values from who have no formal school or only completion of elementary school should be looked at with caution due to low frequencies.
Socioeconomic class compared to knowledge scores yielded a Welch statistic of 0.266 and a significance of 0.767 ( p > 0.05). No further analysis was needed.
The type of social media used compared to knowledge score showed a Welch statistic of 7.175 and significance of < 0.001( p < 0.05). Games Howell determined that Twitter users had significantly higher scores than Facebook (means difference 0.43812, significance of 0.001) and Instagram (means difference 0.69491, significance of 0.001).
Hours spent on social media showed a Welch statistic of 2.531 and significance of 0.044 ( p < 0.05). Post hoc testing showed significantly lower values in those who use social media for 3–4 h compared to 0–2 h (means difference 0.33869, significance of 0.018). No other means from this analysis were significant.
Whether or not a respondent had seen anything on social media about vaccines was not analyzed because there are only 2 categories and therefore the question is noncompliant with the Welch analysis. The influence of vaccine posts on social media had a Welch statistic of 145.202 with a significance of < 0.001 ( p < 0.05). Post hoc testing revealed that those who now perceived their opinion of vaccine of being worse than previously thought had significantly lower scores compared to those who now think vaccines are better (means difference − 6.36712, significance of < 0.001), no influence/change in opinion (means difference − 5.83564, significance of < 0.001) and those who had not seen anything (means difference − 4.70483, significance of < 0.001). Those who think vaccines are better after seeing social media posts had significantly higher scores compared to worsened opinions (as mentioned before), those who were not influenced (means difference 0.53148, significance < 0.001) and those who have not seen anything (means difference 1.66229, significance < 0.001). In addition, those who have not been influenced by posts had significantly higher scores than those who have not seen any posts (means difference 1.13081, significance < 0.001).
Lastly, those trusted for immunization related information and decisions was analyzed and found a Welch statistic of 83.032 with significance of < 0.001 ( p < 0.05). Post hoc analysis showed those who trusted Doctors the most have significantly higher scores than those who trusted the internet (means difference of 5.32139, significance of < 0.001), family (means difference 5.94306, significance < 0.001), and peers (means difference 6.31957, significance of < 0.001). Those who trusted the government the most also had significantly higher scores than internet (means difference 5.13429, significance < 0.001), family (means difference 5.75595, significance of < 0.001) and peers (means difference 6.13247, significance of < 0.001). Trusting of social media should be looked at with caution due to low frequencies.
Depiction of the frequency of belief scores in the sample population can be found in Table 2, found in the supplementary document 2 labeled “Tables”. As noted in the methods, the remaining 6 questions were scored on a two point scale resulting in a belief score from 0 to 12. Scores toward 0 represent negatively skewed beliefs or belief in common myths. Scores toward 12 represent positively skewed beliefs or disbelief in common myths. Scores of 6 represent uncertainness.
The analysis of the demographic questions against the individual’s belief score was completed by Welch and then further analyzed by Games Howell. Explanations of why these tests were chosen can be found in methods. When age was compared with belief score a Welch statistical value of 2.923 and significance of 0.013 ( p < 0.05). Post Hoc revealed 65-year-olds and older had significantly lower scores than 10–24-year-olds (mean difference − 1.37750, significance 0.014) and 24–34 year olds (mean difference − 1.19606, significance 0.047).
Gender analysis showed a Welch statistic of 0.320 with a significance value of 0.728 ( p > 0.05). Post hoc analysis was not examined because there was no significance.
Geographical Welch testing showed a statistic value of 29.212 with a significance of < 0.001( p < 0.05). Due to this value being statically significant, post hoc analysis was examined. North Americans (USA) had significantly lower belief scores compared to Europe (mean difference − 1.47989, significance < 0.001), and Australia/Oceania (means difference − 1.81575, significance < 0.001). North Americans (Other) also showed significantly lower belief scores compared to Europe (means difference − 1.29021, significance < 0.001) and Australia/Oceania (means difference − 1.62607, significance < 0.001). Values from Asia, Africa, and South American should be looked at cautiously because of the low response rate. Antarctica not included in these calculations because there was only one individual who responded.
The analysis of individuals with the highest level of education completed showed a Welch statistic of 17.789 and significance of < 0.001 ( p < 0.05). Post hoc showed that those who completed a professional degree had significantly higher scores than those with master’s degree (mean difference 0.74516, significance of 0.009), bachelor’s degree (means difference 1.10881, significance of < 0.001), associate’s degree (means difference 2.02797, significance of < 0.001), and high school (means difference 1.97009, significance < 0.001). Respondents with master’s degrees were significantly higher scoring than those with associate degrees (means difference of 1.28280, significance < 0.001), and high school (means difference of 1.22493, significance of < 0.001). Those with bachelor’s degrees had significantly higher scores compared to those with associate’s degrees (means difference of 0.91916, significance of < 0.001) and high school (means difference of 0.86128, significance of < 0.001). The values from those individuals that had no formal schooling, or only completion of elementary school, should be looked at cautiously due to low frequencies.
Socioeconomic class, compared to belief scores, resulting in a Welch statistic of 0.028 and a significance of 0.972 ( p > 0.05). No further analysis was needed.
The type of social media users compared to belief score showed a Welch statistic of 8.011 and a significance of < 0.001( p < 0.05). Games Howell determined that Twitter users had significantly higher scores than Facebook (means difference 0.55094, significance of 0.001) and Instagram (means difference 0.98733, significance of < 0.001).
Hours spent on social media showed a Welch statistic of 3.162 and a significance of 0.016 ( p < 0.05). Post hoc testing showed significantly lower values in those who used social media for 3–4 h compared to 0–2 h (means difference 0.39195, significance of 0.034). No other means from this analysis were significant.
Exposure to posts on social media about vaccinations was not analyzed because there were only two categories and therefore, noncompliant with the Welch analysis. The influence of vaccine posts on social media had a Welch statistic of 312.900 with a significance of < 0.001 ( p < 0.05). Post hoc testing revealed that those who now perceived their opinion of vaccines of being worse than previously thought, had significantly lower scores compared to those who now think vaccines are better (means difference − 7.97280, significance of < 0.001), No influence or change in opinion (means difference − 7.27248, significance of < 0.001), and those who had not seen anything (means difference − 4.97992, significance of < 0.001). Those who thought vaccines were better after seeing social media posts had significantly higher scores compared to worsened opinions, as mentioned before, and to those who were not influenced (means difference 0.70031, significance < 0.001), and those who had not seen anything (means difference 2.99288, significance < 0.001). Also, those who had not been influenced by social media posts had significantly higher scores than those who had not seen any posts at all (means difference 2.29256, significance < 0.001).
Finally, those trusted in immunization related information and decisions were analyzed and found a Welch statistic of 150.953 with a significance of < 0.001 ( p < 0.05). Post hoc analysis showed those who trusted doctors the most had significantly higher scores than those who trusted social media (means difference of 6.50713, significance of < 0.001), family (means difference 7.28796, significance < 0.001), and peers (means difference 7.41258, significance of < 0.001). Individuals who trusted the government the most also had significantly higher scores than social media (means difference 6.87265, significance < 0.001), family (means difference 7.65349, significance of < 0.001) and peers (means difference 7.77811, significance of < 0.001). Trusting of social media should be looked at cautiously due to its low frequencies.
The significance found in this study can help us understand who is being influenced by posts about vaccines on social media. It is important to note that the sample in this study was originally started in North America; hence the vast majority of respondents reported residing on this continent. Beyond that, significant values from the statistics should be examined to determine what it means for this research and for further implications.
Mean scores
Overall this study found that respondents were very knowledgeable with a mean knowledge score of 10.4. Very few people had negatively skewed knowledge 0–4 (138 people, 5.7% of the total study population). Further investigation into those people that scored lowest would be able to show greater detail into the minds of those people and where the lack of factual knowledge is coming from or what hurdle needs to be faced.
Respondents had mainly positive beliefs about vaccines with a mean belief score of 9.68, with a standard deviation of 3.14. Few people had negatively skewed beliefs 0–4 (236 people, 9.8% of the total study population). Looking deeper into the individuals that had lower scores would be able to show greater explanation into why their beliefs about vaccines were negative, and how we might be able to change these beliefs.
In a study in 2009, 75.64% of people aged 18–64 were internet users and 74% of users aged 18–24 were social media users (Chou et al). This is important because the high social media use group from that study is now 27–31 years old and could now be making decisions about vaccines for their children.
When looking at ages, we saw that 65 years and older tend to have more negative beliefs than those individuals that are 18–24 and 25–34, with a significant difference of 0.013 ( p < 0.05). Meaning, older people tend to believe the things they see on social media more than someone who is younger. We saw that in the previous research, older people are less likely to use social media [ 4 ], but in this study, those who did use social media scored lower. There were no significant correlations regarding age for knowledge scores.
Gender and socioeconomic status (SES)
A comprehensive research paper by UNICEF in 2013 [ 36 ] titled “Tracking anti-vaccination sentiment in eastern European social media networks” found that females are more likely to discuss developmental disabilities, chemical, toxins and potential side effects whereas males are more likely to discuss conspiracy theories, religion and distrust of the government.
Across all genders and all SES, we saw no significant differences in knowledge or beliefs. This is significant to note because as previous literature and this one found, women are the predominant social media users. Since gender and SES have no impact on vaccine knowledge or beliefs we look deeper into other variables.
For geography, analysis was according to continent lived on and subsequently found statistical significance. North Americans compared to all continents are significantly less knowledgeable about vaccines and had more negatively skewed beliefs. Although this research does not give a reason to why North Americans are less knowledgeable and believe more myths it gives us insight into areas that need to be studied.
There was little data available on Asia, Africa, and South America and none on Antarctica making analysis unreliable and therefore all of which should be disregarded. It is evident that Asia is showing a lower mean knowledge than other areas but without significance due to low frequency. This is paralleled when looking at beliefs. This may be explained by previous research has found that “among people who use the internet, those in developing countries often turn out to be more likely than their counterparts in advanced economies to network via platforms like Facebook and Twitter” [ 33 ]. Further investigation should determine if Asian countries do in fact have lower knowledge of vaccines and why this is. Some things to determine include whether there is a quantifiable difference between continental education, social media, use, cultural perceptions of vaccination, etc.
There is a steady incline in mean knowledge and mean belief score as education level increases. Due to low response frequencies of “no education” and “elementary education” results pertaining to these categories should be disregarded. There are significant differences between all other education levels in an increasing fashion for both knowledge and beliefs. This tells us that those with higher education are in some way or another seeking and finding valid information about vaccines. “Formal schooling adds significant value to innate ability in the form of higher-order cognitive skills crucial to decisions about health” [ 2 ]. This is important in terms of whom to focus on for future vaccine education and propaganda.
Social media platform
A comprehensive research paper titled “Tracking Anti Vaccination Sentiment in Eastern European Social Media Networks” by UNICEF in 2013 [ 36 ] found that vaccine influencers (people or pages that speak publicly about vaccines, both positively and negatively) are most prominent on Facebook and Twitter. This study found that Twitter users are significantly more knowledgeable about vaccines than Facebook or Instagram users and also had more statistically significant positive beliefs about vaccines. Somehow, information is reaching Twitter users but not reaching other forms of social media. Upon further investigation research has showed “information sought from Facebook may be obtained socially (i.e. by asking other users), whereas the information sought on Twitter might be more cognitively based, such as academic or political information that is best gained by reading source materials, for which links are often ‘tweeted’ “[ 22 ].
Time on social media
While the majority of people claimed to only spend 0–2 h on social media daily, those who spent more time, namely 3–4 h, were significantly more knowledgeable about vaccines and had significantly more positive beliefs about vaccines. Although not explored in this study, other studies have found that upwards of 90% of young adults use social media and this number is increasing every year and the majority of this time is spend on smartphones [ 37 ]. Possible reasons for the increased scores may be that those who spend more time on social media could be spending that time reading more deeply into conversations or information. Although it does not appear to be currently studied, there could also be an association between access to a smartphone and the ability to fact check information on the spot.
Vaccine related posts
One of the most interesting takeaways from the data analysis is how vaccine posts have influenced opinions. Those who reported that after seeing vaccine posts they now think vaccines are worse have significantly lower knowledge and belief scores. The opposite is true for those who reported more positive opinions since seeing posts, their scores were significantly higher. The importance of this is that people are able to accurately self-report how these vaccine posts affect them. Those people who see posts about vaccines that make them think vaccines are “bad” have less knowledge or their knowledge has been changed from correct to incorrect with these posts.
A European study found that over 40% of respondents had some degree of “negative feelings about vaccine safety” [ 28 ]. These “fear” posts are typically full of myths, although not inherently known to be myths by the reader, about vaccines and as a result people became more likely to believe the myths. While it cannot be inferred why this is happening it is an interesting statistic. The presence of this significance lets health/vaccine promoters, such as government; know that the vaccine posts with valid knowledge and promotion are working. Those respondents that reported they were not influenced by social media posts, had lower belief scores compared to those who said they were positively influenced by social media posts. Those who reported they were not influenced had similar knowledge scores to those who were positively influenced. This may be due to the ability to weed through information to find facts versus fiction.
The internet is now the easiest and most accessible way to get information. A google search from the United States of America for the term “vaccination” yielded 71% anti-vaccination pages and only 29% pro vaccination pages [ 25 ]. Many of these anti-vaccinations sites claimed that vaccines contain “poisons” and were damaging to the human body [ 25 ]. “In many cases, [those who perceive vaccines as harmful] may become the only individuals who voice their opinions [ 32 ]. This allows for a greater propagation of anti-vax information rather than pro-vaccination and factual knowledge. Googling information about the MMR vaccine and autism only 51% of the search results yielded correct information [ 41 ]. Not only is it important to understand the diversity of search results but also the quality of the websites appearing. Many of “sites masquerading as official scientific sites, some web users may not question the veracity of such material” [ 12 ]. Although this study does not specifically focus on the internet, social media allows for easy sharing of not only opinions but also links to some of these “pseudo-science” websites.
Trusted source
Lastly, the analysis of who people trust most with their vaccine related information and decisions. Literature review revealed a study that found that parents who used the internet to get their information about vaccines were more likely to think children do not benefit from vaccines [ 24 ]. It is comforting to know that the vast majority of respondent in this study do in fact rely on the information they receive from their doctor and government. While many anti-vax campaigns are grounded on the fact that authorities are misconstruing vaccine information, the idea of big pharma, it is evident that that ideal is not being taken up by social media users. Those who trust the government and doctors with their information were significantly more knowledgeable about vaccine and more positive beliefs facts than those who trust another group. While it is known that many anti-vaxxers rely on social media to disseminate their message, very few people trust social media as a source. This is very good news for doctors whose patients seek information from them and government run websites such as health departments and health organizations.
Limitations
Some limitations faced by this research include the cross sectional nature of this study. Cross sectional studies cannot infer causation, only correlations. It also limits this survey to only surface information and not important individual details. In addition, a limitation may be that demographics were not cross analyzed. This means that while it was found that professionals had the highest knowledge score this research does not delve into the gender, location, age, etc. of these people. This same information could be analyzed in this way for further investigation. It is also important to note that this information is limited by some frequencies being too low for confidence. These areas would ideally have to be isolated and looked at again with higher number of participants. These categories were mentioned in the discussion and results stating they should be disregarded.
This study is also limited by the snowball sampling method. This limits the study by which participants decided to share, and where the majority of their friends are located, as seen by the North American predominance. While great caution was taken when constructing this survey to be nonjudgmental, some valuable information is lost in the fact that respondents were not asked their vaccine practices.
As with all online surveys, no interviewer was present. Respondents were not able to clarify answers that they felt needed explanation. Without an interviewer there is no probing into deeper answers. Information this study found could be used in the future for interviewer based research to determine a more in depth understanding of those who lacked vaccine knowledge. Another limitation of being an online survey is that there is no accountability for answers. Respondents could have clicked through the survey to complete all the questions as quickly as possible without regard for what the answer choices were.
The information portrayed in this research by the authors favors scientific fact as described by other journal articles and not personal opinions/bias. Every effort was made not to use incriminating or offensive language and statements in the creation of the survey. This core of this research was completed by the authors when they were medical students. Saint James School of Medicine ethics committee approved this research and all of its modalities. Survey platform was provided by Saint James School of Medicine.
As social media continues to grow exponentially, it can be expected that anti-vaxxers will further spread their messages across these platforms. While this research does not delve into the totality and extent of the anti-vax movement on social media it does provide some insightful information. As mentioned previously, it is possible for governments and doctors to use this information to intervene and correct false information about vaccines and their safety. Given that trust in doctors resulted in significantly higher knowledge and belief scores, it is imperative that physicians create a trusting environment and relationship with their patients and guardians of pediatric patients. This also tells us that the information patients are getting from their doctors and the conversations they are having are influencing vaccine decision making in a positive way. What was not studied, and would be beneficial to investigate going forward, is whether doctors have the ability to change a patient’s perspective on vaccines once they have been exposed to negatively skewed vaccine information. It is also evident that those who trust the internet with their vaccine information are exposed to more misinformation. There may be an avenue for addressing misinformation on the internet through this trusted source and that could be via physician social media influencers. We have started to see the importance of this avenue with the prevalence of COVID-19. Social media has create an easily accessibly way to reach credible sources such as CDC and WHO, who made their presence known during the height of the pandemic through social media campaigns [ 7 ]. This appears to be especially important for direct intervention on Facebook, where the majority of social media use is, and unfortunately lower rates of knowledge and more negatively skewed beliefs. In North America, actions should be taken to combat the misinformation and myths that are reaching its population more than anywhere else in the world.
Further research is recommended to understand why some countries, age groups, social network users, etc. are not getting adequate information on vaccines. Future research would benefit from using a structured interviewer based approach to allow for expansion and clarification of answers. Further evaluation of the data collected here could yield more in-depth understanding of demographics. As mentioned before this research did not cross analyze demographics.
Availability of data and materials
Raw and descriptive data is available from openICPSR.org project ID openicpsr-120505.
Abbreviations
Anti- vaccinator / Vaccine denier
Human Papilloma Virus vaccine
Measles Mumps Rubella vaccine
United Nations International Children’s Emergency Fund
Statistical package for social sciences
Socioeconomic status
Armitage R. Online ‘anti-vax’campaigns and COVID-19: censorship is not the solution. Public Health. 2021;190:e29. https://doi.org/10.1016/j.puhe.2020.12.005 .
Article CAS PubMed Google Scholar
Baker DP, Leon J, Smith Greenaway EG, Collins J, Movit M. The education effect on population health: a reassessment. Popul Dev Rev. 2011;37(2):307–32. https://doi.org/10.1111/j.1728-4457.2011.00412.x .
Article PubMed PubMed Central Google Scholar
Baltar F, Brunet I. Social research 2.0: virtual snowball sampling method using Facebook. Internet research; 2012.
Google Scholar
Bell C, Fausset C, Farmer S, Nguyen J, Harley L, Fain WB. Examining social media use among older adults. In: Proceedings of the 24th ACM conference on hypertext and social media; 2013. p. 158–63.
Chapter Google Scholar
Benoit SL, Mauldin R. The “anti-vax” movement: a quantitative report on vaccine beliefs and knowledge across social media. Ann Arbor: Inter-university Consortium for Political and Social Research [distributor]; 2020. https://doi.org/10.3886/E120505V2
Betsch C, Brewer NT, Brocard P, Davies P, Gaissmaier W, Haase N, et al. Opportunities and challenges of web 2.0 for vaccination decisions. Vaccine. 2012;30(25):3727–33. https://doi.org/10.1016/j.vaccine.2012.02.025 .
Article PubMed Google Scholar
Brindha MD, Jayaseelan R, Kadeswara S. Social media reigned by information or misinformation about COVID-19: a phenomenological study. Alochana Chakra J. 2020;9(5):585–602.
Brunson EK. The impact of social networks on parents’ vaccination decisions. Pediatrics. 2013;131:e1397 peds-2012.
Article Google Scholar
Chou WYS, Hunt YM, Beckjord EB, Moser RP, Hesse BW. Social media use in the United States: implications for health communication. J Med Internet Res. 2009;11(4):e48. https://doi.org/10.2196/jmir.1249 .
Chow MYK, Danchin M, Willaby HW, Pemberton S, Leask J. Parental attitudes, beliefs, behaviours and concerns towards childhood vaccinations in Australia: a national online survey. Aust Fam Physician. 2017;46(3):145.
Cvjetkovic SJ, Jeremic VL, Tiosavljevic DV. Knowledge and attitudes toward vaccination: a survey of Serbian students. J Infect Public Health. 2017;10(5):649–56. https://doi.org/10.1016/j.jiph.2017.05.008 .
Davies P, Chapman S, Leask J. Antivaccination activists on the world wide web. Arch Dis Child. 2002;87(1):22–5. https://doi.org/10.1136/adc.87.1.22 .
Article CAS PubMed PubMed Central Google Scholar
Duggan M, Brenner J. The demographics of social media users, 2012, vol. 14. Washington, DC: Pew Research Center’s Internet & American Life Project; 2013.
Dunn AG, Leask J, Zhou X, Mandl KD, Coiera E. Associations between exposure to and expression of negative opinions about human papillomavirus vaccines on social media: an observational study. J Med Internet Res. 2015;17(6):e144. https://doi.org/10.2196/jmir.4343 .
Durbach N. Bodily matters: the anti-vaccination movement in England, 1853–1907: Duke University Press; 2005. https://doi.org/10.1215/9780822386506 .
Fagerland MW, Sandvik L. Performance of five two-sample location tests for skewed distributions with unequal variances. Contemp Clin Trials. 2009;30(5):490–6. https://doi.org/10.1016/j.cct.2009.06.007 .
Feleszko, W., Lewulis, P., Czarnecki, A., & Waszkiewicz, P.. Flattening the curve of COVID-19 vaccine rejection—a global overview. 2020. Available at SSRN.
Book Google Scholar
Fox S, Jones S. The social life of health information. Washington, DC: Pew internet & American life project; 2009.
Freed GL, Clark SJ, Butchart AT, Singer DC, Davis MM. Parental vaccine safety concerns in 2009. Pediatrics. 2010;125:654 peds-2009.
Fricker RD, Schonlau M. Advantages and disadvantages of internet research surveys: evidence from the literature. Field Methods. 2002;14(4):347–67. https://doi.org/10.1177/152582202237725 .
Hilton A, Armstrong R. Statnote 6: post-hoc ANOVA tests; 2006.
Hughes DJ, Rowe M, Batey M, Lee A. A tale of two sites: twitter vs. Facebook and the personality predictors of social media usage. Comput Hum Behav. 2012;28(2):561–9. https://doi.org/10.1016/j.chb.2011.11.001 .
Johnson, T. P.. Snowball sampling: introduction. 2014. Wiley StatsRef : Statistics Reference Online.
Jones AM, Omer SB, Bednarczyk RA, Halsey NA, Moulton LH, Salmon DA. Parents’ source of vaccine information and impact on vaccine attitudes, beliefs, and nonmedical exemptions. Adv Prev Med. 2012;2012:1–8. https://doi.org/10.1155/2012/932741 .
Kata A. A postmodern Pandora’s box: anti-vaccination misinformation on the internet. Vaccine. 2010;28(7):1709–16. https://doi.org/10.1016/j.vaccine.2009.12.022 .
Kata A. Anti-vaccine activists, web 2.0, and the postmodern paradigm–an overview of tactics and tropes used online by the anti-vaccination movement. Vaccine. 2012;30(25):3778–89. https://doi.org/10.1016/j.vaccine.2011.11.112 .
Khubchandani J, Sharma S, Price JH, Wiblishauser MJ, Sharma M, Webb FJ. COVID-19 vaccination hesitancy in the United States: a rapid national assessment. J Community Health. 2021;46(2):270–7. https://doi.org/10.1007/s10900-020-00958-x .
Larson HJ, de Figueiredo A, Xiahong Z, Schulz WS, Verger P, Johnston IG, et al. The state of vaccine confidence 2016: global insights through a 67-country survey. EBioMedicine. 2016;12:295–301. https://doi.org/10.1016/j.ebiom.2016.08.042 .
Massey, E.. A sermon against the dangerous and sinful practice of inoculation . 1722. William Meadows.
McCauley MM, Kennedy A, Basket M, Sheedy K. Exploring the choice to refuse or delay vaccines: a national survey of parents of 6-through 23-month-olds. Acad Pediatr. 2012;12(5):375–83. https://doi.org/10.1016/j.acap.2012.06.007 .
Mitra T, Counts S, Pennebaker JW. Understanding anti-vaccination attitudes in social media. In: ICWSM; 2016. p. 269–78.
Poland GA, Jacobson RM. Understanding those who do not understand: a brief review of the anti-vaccine movement. Vaccine. 2001;19(17–19):2440–5. https://doi.org/10.1016/S0264-410X(00)00469-2 .
Poushter J, Bishop C, Chwe H. Social media use continues to rise in developing countries but plateaus across developed ones. Pew Res Cent. 2018;22:2–19.
Soares P, Rocha JV, Moniz M, Gama A, Laires PA, Pedro AR, et al. Factors associated with COVID-19 vaccine hesitancy. Vaccines. 2021;9(3):300. https://doi.org/10.3390/vaccines9030300 .
Tomarken AJ, Serlin RC. Comparison of ANOVA alternatives under variance heterogeneity and specific noncentrality structures. Psychol Bull. 1986;99(1):90–9. https://doi.org/10.1037/0033-2909.99.1.90 .
UNICEF. Tracking anti vaccination sentiment in eastern European social media networks. New York: UNICEF; 2013.
Villanti AC, Johnson AL, Ilakkuvan V, Jacobs MA, Graham AL, Rath JM. Social media use and access to digital technology in US young adults in 2016. J Med Internet Res. 2017;19(6):e196. https://doi.org/10.2196/jmir.7303 .
Weigmann K. An injection of confidence: scientists explore new and old methods to counter anti-vaccine propaganda and overcome vaccine hesitancy so as to increase vaccination rates. EMBO Rep. 2017;18(1):21–4. https://doi.org/10.15252/embr.201643589 .
World Health Organization. Considerations regarding consent in vaccinating children and adolescents between 6 and 17 years old (No. WHO/IVB/14.04): World Health Organization; 2014.
Yaqub O, Castle-Clarke S, Sevdalis N, Chataway J. Attitudes to vaccination: a critical review. Soc Sci Med. 2014;112:1–11. https://doi.org/10.1016/j.socscimed.2014.04.018 .
Zimmerman RK, Wolfe RM, Fox DE, Fox JR, Nowalk MP, Troy JA, et al. Vaccine criticism on the world wide web. J Med Internet Res. 2005;7(2). https://doi.org/10.2196/jmir.7.2.e17 .
Download references
Acknowledgements
Dr. Branka Filipovich from Saint James School of Medicine for research guidance and draft editing. Dr. Zarina Merchant for supervising the survey portion of this research.
There is no funding associated with this project.
Author information
Authors and affiliations.
Saint James School of Medicine, Park Ridge, Illinois, USA
Staci L Benoit & Rachel F. Mauldin
You can also search for this author in PubMed Google Scholar
Contributions
SB; Lead author, designed analytic scoring/method and data analyst. RM; co-author, co-conceived experimental idea, coordination of timeline, data collection. All authors have reviewed and approved the manuscript.
Corresponding author
Correspondence to Staci L Benoit .
Ethics declarations
Ethics approval and consent to participate.
This research was approved by the ethics committee of Saint James School of Medicine. This research included a consent page present at the beginning of the survey which included consent to participate. Participation was limited to those over 18 years of age. Failure to consent resulted in termination of the remainder of the survey.
Consent for publication
Not applicable.
Competing interests
The authors declare that they have no competing interests.
Additional information
Publisher’s note.
Springer Nature remains neutral with regard to jurisdictional claims in published maps and institutional affiliations.
Supplementary Information
Additional file 1..
Survey. Anti- Vax research survey. This file includes the questions and multiple choice options that were used to build the research’s SurveyMonkey Survey.
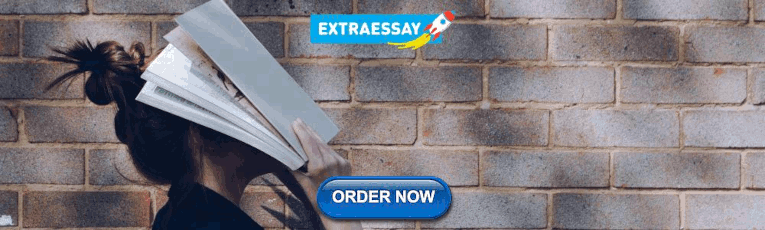
Additional file 2: Table 1.
Frequency of Knowledge Scores 0-12 with percent of total sample population. Table 2. Frequency of Belief scores 0-12 with percent of total sample population.
Rights and permissions
Open Access This article is licensed under a Creative Commons Attribution 4.0 International License, which permits use, sharing, adaptation, distribution and reproduction in any medium or format, as long as you give appropriate credit to the original author(s) and the source, provide a link to the Creative Commons licence, and indicate if changes were made. The images or other third party material in this article are included in the article's Creative Commons licence, unless indicated otherwise in a credit line to the material. If material is not included in the article's Creative Commons licence and your intended use is not permitted by statutory regulation or exceeds the permitted use, you will need to obtain permission directly from the copyright holder. To view a copy of this licence, visit http://creativecommons.org/licenses/by/4.0/ . The Creative Commons Public Domain Dedication waiver ( http://creativecommons.org/publicdomain/zero/1.0/ ) applies to the data made available in this article, unless otherwise stated in a credit line to the data.
Reprints and permissions
About this article
Cite this article.
Benoit, S.L., Mauldin, R.F. The “anti-vax” movement: a quantitative report on vaccine beliefs and knowledge across social media. BMC Public Health 21 , 2106 (2021). https://doi.org/10.1186/s12889-021-12114-8
Download citation
Received : 03 August 2020
Accepted : 27 October 2021
Published : 17 November 2021
DOI : https://doi.org/10.1186/s12889-021-12114-8
Share this article
Anyone you share the following link with will be able to read this content:
Sorry, a shareable link is not currently available for this article.
Provided by the Springer Nature SharedIt content-sharing initiative
- Social media
- Vaccine denier
- Social network
BMC Public Health
ISSN: 1471-2458
- Submission enquiries: [email protected]
- General enquiries: [email protected]

Vaccine breakthrough means no more chasing strains
Scientists at UC Riverside have demonstrated a new, RNA-based vaccine strategy that is effective against any strain of a virus and can be used safely even by babies or the immunocompromised.
Every year, researchers try to predict the four influenza strains that are most likely to be prevalent during the upcoming flu season. And every year, people line up to get their updated vaccine, hoping the researchers formulated the shot correctly.
The same is true of COVID vaccines, which have been reformulated to target sub-variants of the most prevalent strains circulating in the U.S.
This new strategy would eliminate the need to create all these different shots, because it targets a part of the viral genome that is common to all strains of a virus. The vaccine, how it works, and a demonstration of its efficacy in mice is described in a paper published today in the Proceedings of the National Academy of Sciences .
"What I want to emphasize about this vaccine strategy is that it is broad," said UCR virologist and paper author Rong Hai. "It is broadly applicable to any number of viruses, broadly effective against any variant of a virus, and safe for a broad spectrum of people. This could be the universal vaccine that we have been looking for."
Traditionally, vaccines contain either a dead or modified, live version of a virus. The body's immune system recognizes a protein in the virus and mounts an immune response. This response produces T-cells that attack the virus and stop it from spreading. It also produces "memory" B-cells that train your immune system to protect you from future attacks.
The new vaccine also uses a live, modified version of a virus. However, it does not rely on the vaccinated body having this traditional immune response or immune active proteins -- which is the reason it can be used by babies whose immune systems are underdeveloped, or people suffering from a disease that overtaxes their immune system. Instead, this relies on small, silencing RNA molecules.
"A host -- a person, a mouse, anyone infected -- will produce small interfering RNAs as an immune response to viral infection. These RNAi then knock down the virus," said Shouwei Ding, distinguished professor of microbiology at UCR, and lead paper author.
The reason viruses successfully cause disease is because they produce proteins that block a host's RNAi response. "If we make a mutant virus that cannot produce the protein to suppress our RNAi, we can weaken the virus. It can replicate to some level, but then loses the battle to the host RNAi response," Ding said. "A virus weakened in this way can be used as a vaccine for boosting our RNAi immune system."
When the researchers tested this strategy with a mouse virus called Nodamura, they did it with mutant mice lacking T and B cells. With one vaccine injection, they found the mice were protected from a lethal dose of the unmodified virus for at least 90 days. Note that some studies show nine mouse days are roughly equivalent to one human year.
There are few vaccines suitable for use in babies younger than six months old. However, even newborn mice produce small RNAi molecules, which is why the vaccine protected them as well. UC Riverside has now been issued a US patent on this RNAi vaccine technology.
In 2013, the same research team published a paper showing that flu infections also induce us to produce RNAi molecules. "That's why our next step is to use this same concept to generate a flu vaccine, so infants can be protected. If we are successful, they'll no longer have to depend on their mothers' antibodies," Ding said.
Their flu vaccine will also likely be delivered in the form of a spray, as many people have an aversion to needles. "Respiratory infections move through the nose, so a spray might be an easier delivery system," Hai said.
Additionally, the researchers say there is little chance of a virus mutating to avoid this vaccination strategy. "Viruses may mutate in regions not targeted by traditional vaccines. However, we are targeting their whole genome with thousands of small RNAs. They cannot escape this," Hai said.
Ultimately, the researchers believe they can 'cut and paste' this strategy to make a one-and-done vaccine for any number of viruses.
"There are several well-known human pathogens; dengue, SARS, COVID. They all have similar viral functions," Ding said. "This should be applicable to these viruses in an easy transfer of knowledge."
- Cold and Flu
- Infectious Diseases
- Immune System
- HIV and AIDS
- Flu vaccine
- Global spread of H5N1 in 2006
- Global spread of H5N1
- Acupuncture
- MMR vaccine
Story Source:
Materials provided by University of California - Riverside . Original written by Jules Bernstein. Note: Content may be edited for style and length.
Journal Reference :
- Gang Chen, Qingxia Han, Wan-Xiang Li, Rong Hai, Shou-Wei Ding. Live-attenuated virus vaccine defective in RNAi suppression induces rapid protection in neonatal and adult mice lacking mature B and T cells . Proceedings of the National Academy of Sciences , 2024; 121 (17) DOI: 10.1073/pnas.2321170121
Cite This Page :
Explore More
- Two Species Interbreeding Created New Butterfly
- Warming Antarctic Deep-Sea and Sea Level Rise
- Octopus Inspires New Suction Mechanism for ...
- Cities Sinking: Urban Populations at Risk
- Puzzle Solved About Ancient Galaxy
- How 3D Printers Can Give Robots a Soft Touch
- Combo of Multiple Health Stressors Harming Bees
- Methane Emission On a Cold Brown Dwarf
- Remarkable Memories of Mountain Chickadees
- Predicting Future Marine Extinctions
Trending Topics
Strange & offbeat.
Scripps Research reports progress toward creating vaccine against the harmful ‘zombie’ drug xylazine
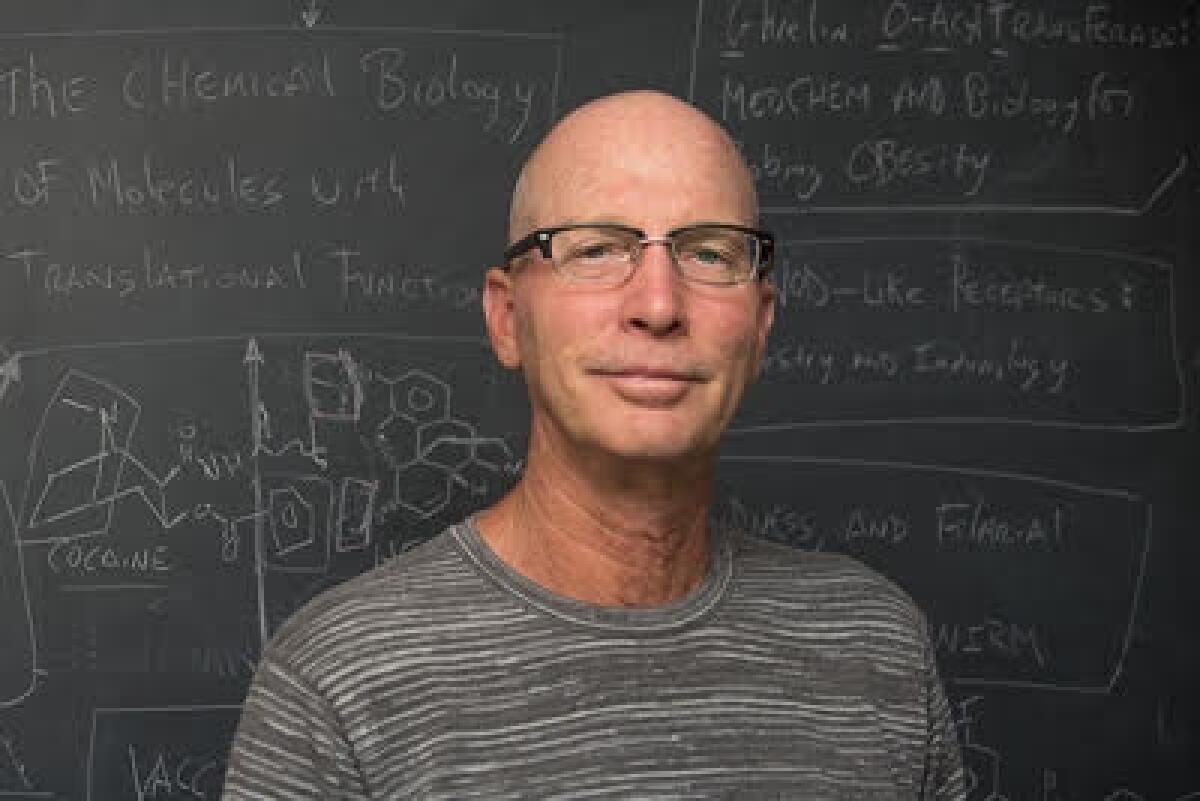
An experimental vaccine blocked the toxic effects of the drug — which is frequently added to heroin and illicit fentanyl — in experiments conducted on rodents, according to research published this month
- Show more sharing options
- Copy Link URL Copied!
Scripps Research in La Jolla says it has taken a promising step toward developing a vaccine to fight the effects of xylazine, an animal tranquilizer that’s illicitly added to fentanyl and heroin, triggering a rise in overdose deaths.
The institute’s experimental vaccine blocked xylazine’s toxic effect in experiments conducted on rodents, according to a paper published earlier this month in the journal Chemical Communications. It works by stimulating the immune system to create antibodies that reduce the level of xylazine in the blood stream, the institute said.
Veterinarians regularly use xylazine to sedate or calm everything from sheep and cattle to cats and dogs before surgery or while conducting diagnostic tests. It’s been approved for that purpose by the U.S. Food and Drug Administration. But it is not approved for use in humans because it can slow breathing and heart rates and produce dangerously low blood-pressure levels.
Scientists say xylazine, a non-opioid sedative, also can cause skin lesions and wounds that don’t heal and sometimes lead to the need to amputate parts of legs or arms.
Xyaline is often illicitly added to fentanyl and other drugs to lengthen the feeling of euphoria they can give, scientists say. It has taken on the nickname “zombie drug.”
“There is currently no remedy for xylazine poisoning other than supportive care, thus, we believe our research efforts and the data we have provided will pave the way for an effective treatment in humans,” Kim D. Janda, the Scripps chemist who is leading the research, said in a statement.
The precise number of human deaths caused by the addition of xylazine to fentanyl and other drugs is not known. But the Journal of the American Medical Association reported in January that at least 43 states reported deaths related to xylazine, which also is known as “tranq dope.”
Scripps Research is deeply involved in vaccine research and made significant contributions to the COVID-19 vaccines developed by Moderna and by Pfizer and BioNTech.
Get Essential San Diego, weekday mornings
Get top headlines from the Union-Tribune in your inbox weekday mornings, including top news, local, sports, business, entertainment and opinion.
You may occasionally receive promotional content from the San Diego Union-Tribune.
More from this Author
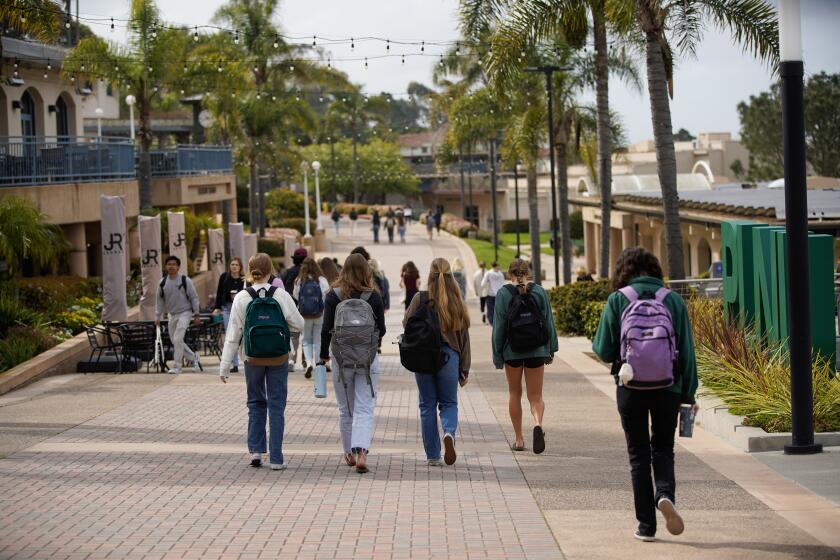
Once again, Point Loma Nazarene University’s acceptance of gay people is called into question by its own students
April 20, 2024
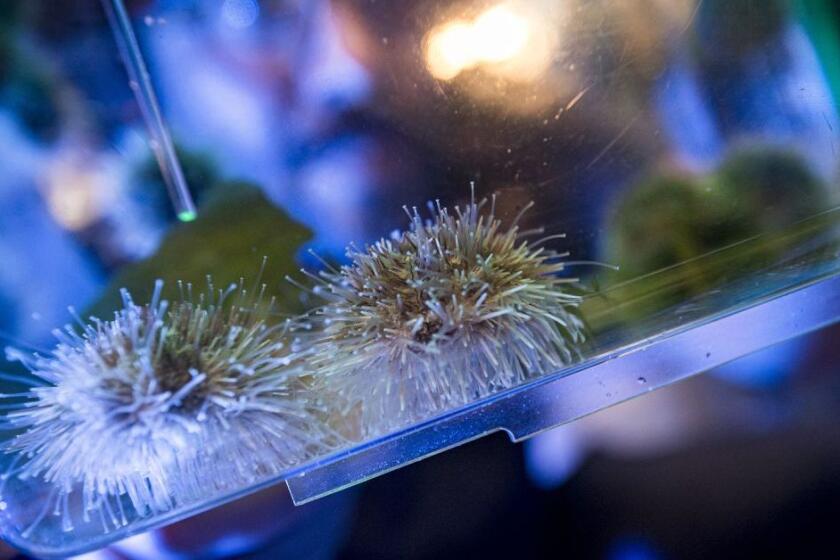
What’s polluting the oceans? UC San Diego to lead large study of contaminants’ source, fate and health impacts
April 16, 2024
San Diego County weather will finally turn warmer after long cool stretch
April 15, 2024
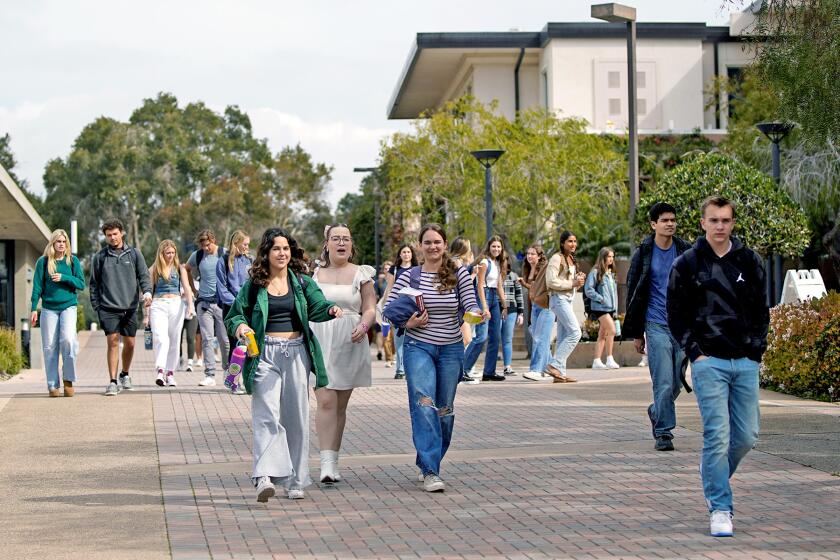
Refuge for the religious, or prestigious STEM powerhouse? Point Loma Nazarene University, at a crossroads, frets over its future
April 14, 2024
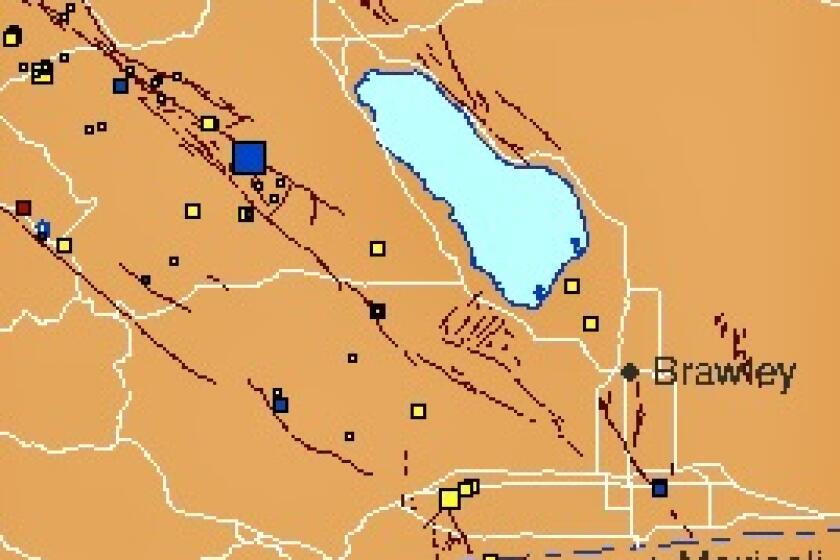
3.8 earthquake near Borrego Springs briefly shakes parts of San Diego County
April 13, 2024
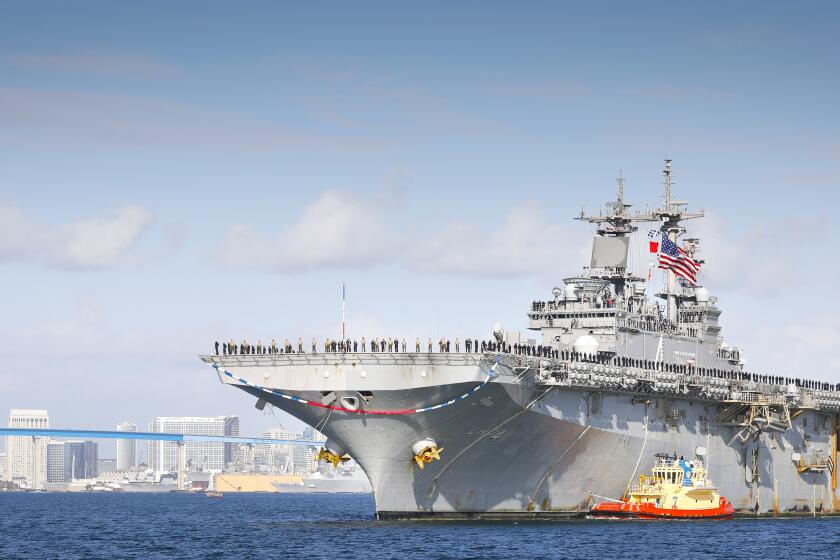
Mechanical problems force USS Boxer to return from deployment 10 days after it sailed out of San Diego Bay
April 12, 2024
More in this section

Hiring is hot for government and health care jobs. These sectors boosted San Diego’s employment gains
The San Diego region gained about 4,400 jobs in March. The demand for registered nurses leads local job openings.
April 19, 2024
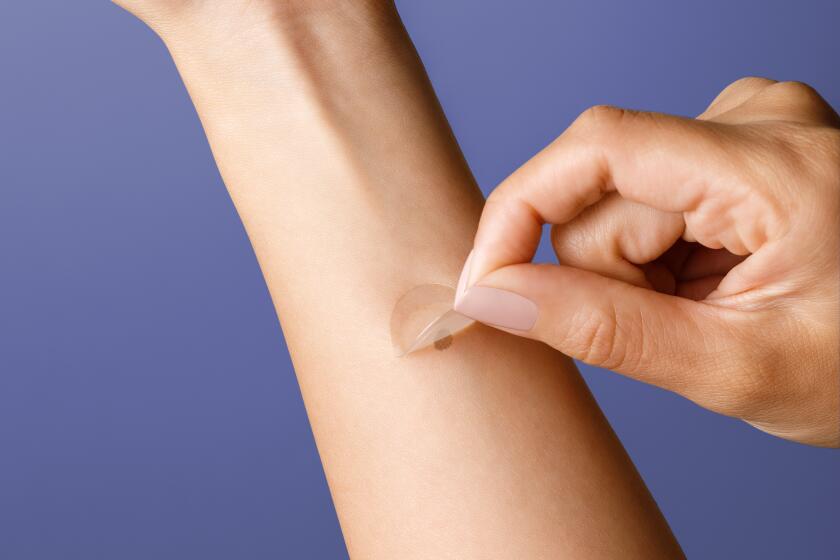
San Diego biotech company making melanoma test patch cuts 56% of its workforce
San Diego-based DermTech is also exploring the possibility of a merger, acquisition, sale of assets, licensing or other transaction.
April 18, 2024
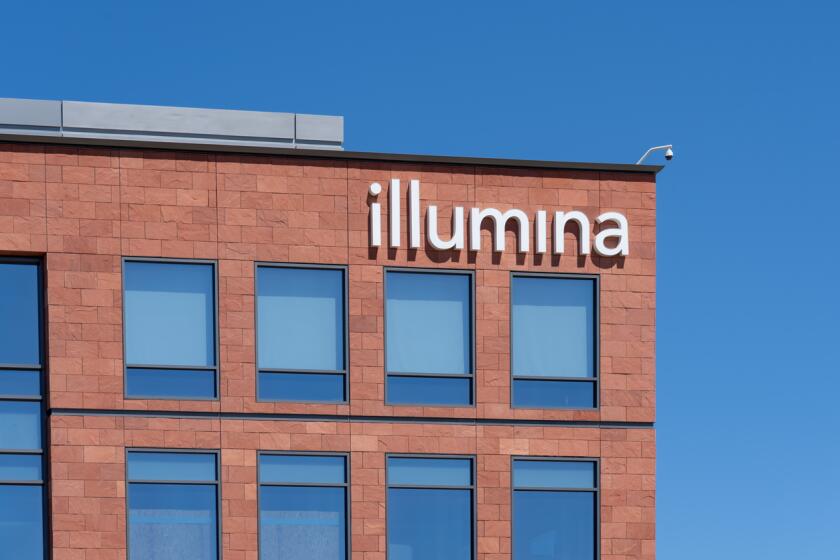
Illumina gets two new executives as the San Diego biotech revamps its C-suite
Illumina’s chief financial officer since early 2023 is leaving the company, and a new one is already lined up
April 10, 2024
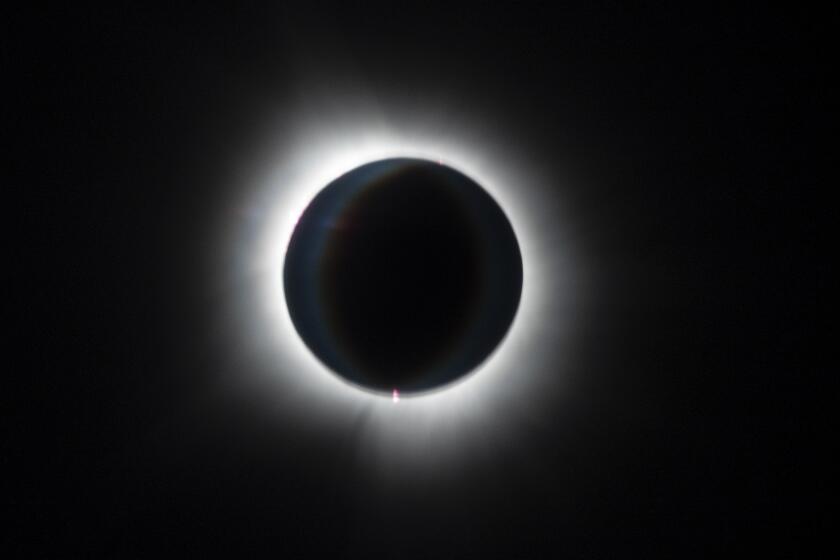
As more than half the sun slips into eclipse, oohs and ahs fill the air across San Diego County
The skies turn out to be much clearer than expected, enabling people to see a rare phenomenon that never disappoints
April 8, 2024
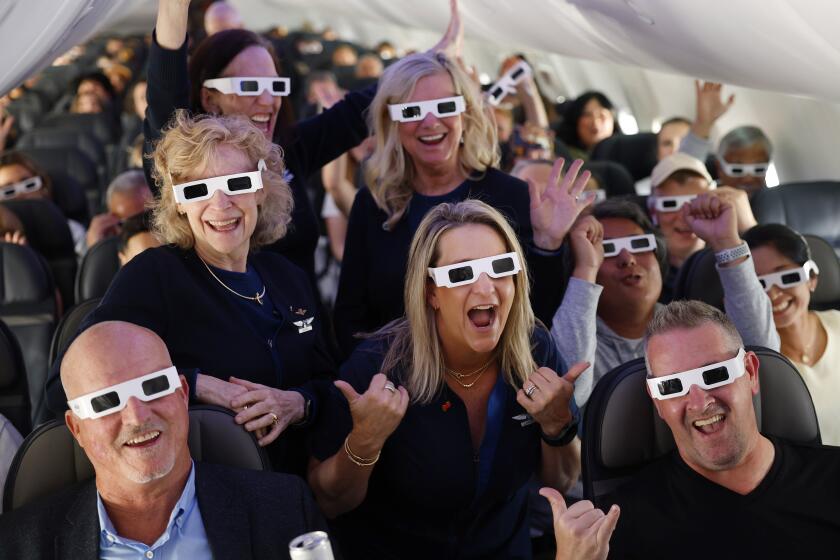
San Diego travelers view eclipse from 33,000 feet
ABOARD FLIGHT AS322 — As tens of thousands of people flocked to cities from Texas to Maine for a chance to see the total solar eclipse, others took a chance on one of the rarest views of the phenomenon: out the window of a jetliner 33,000 feet in the air.

More rocket launches could light up the San Diego sky as SpaceX builds out satellite network
Space X asks the California Coastal Commission to OK as many as 36 launches annually from Vandenberg Space Force Base
April 6, 2024
- Share full article
Advertisement
Supported by
Global Health
The Push for a Better Dengue Vaccine Grows More Urgent
A public research institute in Brazil has proved a new shot protects against the disease, but can’t make it fast enough to stop the huge outbreak sweeping Latin America.

By Stephanie Nolen
Stephanie Nolen has been covering the growing health threat from mosquitoes and the scientific quest for new solutions.
The outbreak of dengue fever that has unfolded in Latin America over the past three months is staggering in its scale — a million cases in Brazil in a matter of weeks, a huge spike in Argentina, a state of emergency declared in Peru and now another in Puerto Rico.
It forewarns of a changing landscape for the disease. The mosquitoes that spread dengue thrive in densely populated cities with weak infrastructure, and in warmer and wetter environments — the type of habitat that is expanding quickly with climate change.
More than 3.5 million cases of dengue have been confirmed by governments in Latin America in the first three months of 2024, compared with 4.5 million in all of 2023. There have been more than 1,000 deaths so far this year. The Pan-American Health Organization is warning that this may be the worst year for dengue ever recorded .
The rapidly shifting disease landscape needs new solutions, and researchers in Brazil delivered the lone shred of good news in this story with the recent announcement that a clinical trial of a new dengue vaccine, delivered in a single shot, had provided strong protection against the disease .
There are two existing vaccines for dengue, but one is an expensive two-shot regimen, while the other can be given only to people who have already had a dengue infection.
The new one-shot vaccine uses live, weakened forms of all four strains of the dengue virus. It was created by scientists at the National Institutes of Health in the United States and licensed for development by the Instituto Butantan, a huge public research institute in São Paulo.
Butantan will make the vaccine. It already produces most of the immunizations used in Brazil, and has the capacity to make tens of millions of doses of this new one. The institute plans to submit the dengue vaccine to Brazil’s regulatory agency for approval in the next few months and could begin producing it next year.
But that won’t help with this outbreak and, by the time the production gears up and a national rollout gets started, it may not be enough to help with the next one, either; dengue typically surges in three- or four-year cycles.
And it won’t necessarily be of help to the rest of Latin America: Butantan will only make the vaccine for Brazil. The multinational drug company Merck & Co., which also licensed the NIH technology, is developing a related vaccine which will be sold in the rest of the world; the efficacy of that vaccine has not yet been tested in a late-stage clinical trial.
And there is, of course, demand for a dengue vaccine beyond the Americas: Mosquitoes are spreading the disease to Croatia, Italy, California and other regions that haven’t seen it before. Places used to handling mild outbreaks now face record-breaking ones: Bangladesh had 300,000 cases last year.
Dengue is commonly known by the name breakbone fever, after the excruciating joint pain it causes. Not everybody experiences that pain: Three-quarters of people infected with dengue don’t have any symptoms at all, and among those who do, most cases resemble only a mild flu.
But about 5 percent of people who become sick will progress to what’s called severe dengue. Plasma, the protein-rich fluid component of blood, can start to leak out of blood vessels, causing patients to go into shock or have organ failure.
When patients with severe dengue are treated with blood transfusions and intravenous fluids, the mortality rate tends to be between 2 and 5 percent. But when they don’t get treatment — because they don’t realize it’s dengue and don’t seek treatment quickly enough, or because health centers are overwhelmed — the mortality rate is 15 percent.
In Brazil, the current dengue outbreak is hitting children hardest; those under 5 have the highest mortality rate of any age group, followed by those age 5 to 9. Adolescents between 10 and 14 have the highest number of confirmed cases, according to the Instituto Oswaldo Cruz , a national public health research center.
As clinics began to be overwhelmed with dengue patients in January, the Brazilian government bought the entire global stock of a Japanese-made vaccine for dengue called Qdenga. Public health nurses are delivering it to children ages 6 to 16, but there will be enough vaccine to fully vaccinate only 3.3 million of Brazil’s 220 million people this year.
This big national effort will protect a few million children, but it won’t contribute anything to its herd immunity.
Qdenga is not cheap: It’s about $115 per dose in Europe and $40 in Indonesia. Brazil is paying $19 per dose, having negotiated a lower price for its huge purchase.
Takeda Pharmaceuticals, which makes Qdenga, announced a deal last month with Biological E, a large Indian generic drug maker, to license and produce up to 50 million doses a year, part of a race to accelerate production. The Indian vaccine should cost considerably less. But Biological E is unlikely to have regulatory approval to market it before 2030; it’s a slow process that involves transferring technology, setting up a production line and getting a new version of even a well-known product approved by regulators.
Dengue costs Brazil at least $1 billion a year in health care treatment and lost productivity. And that figure doesn’t take into account the human suffering involved.
The fact that there are four different strains of the dengue virus complicates more than the process of making a vaccine: The potentially fatal form of the disease is more common when patients have a second infection, with a different strain than they had the first time. Qdenga protects against all four strains of dengue, and the hope is that the new Butantan vaccine does, too, although the data released so far shows it tested against only the two types that were circulating during the first part of the trial; more results are expected in June.
Millions more people will have been exposed to dengue when this outbreak finally passes. But they’re going to need that new vaccine more urgently than ever.
Stephanie Nolen is a global health reporter for The Times. More about Stephanie Nolen

An official website of the United States government
The .gov means it’s official. Federal government websites often end in .gov or .mil. Before sharing sensitive information, make sure you’re on a federal government site.
The site is secure. The https:// ensures that you are connecting to the official website and that any information you provide is encrypted and transmitted securely.
- Publications
- Account settings
Preview improvements coming to the PMC website in October 2024. Learn More or Try it out now .
- Advanced Search
- Journal List
- Front Microbiol
Impact of Vaccines; Health, Economic and Social Perspectives
Charlene m. c. rodrigues.
1 Department of Zoology, University of Oxford, Oxford, United Kingdom
2 Department of Paediatric Infectious Diseases, St George’s University Hospitals NHS Foundation Trust, London, United Kingdom
Stanley A. Plotkin
3 Department of Pediatrics, University of Pennsylvania, Philadelphia, PA, United States
In the 20th century, the development, licensing and implementation of vaccines as part of large, systematic immunization programs started to address health inequities that existed globally. However, at the time of writing, access to vaccines that prevent life-threatening infectious diseases remains unequal to all infants, children and adults in the world. This is a problem that many individuals and agencies are working hard to address globally. As clinicians and biomedical scientists we often focus on the health benefits that vaccines provide, in the prevention of ill-health and death from infectious pathogens. Here we discuss the health, economic and social benefits of vaccines that have been identified and studied in recent years, impacting all regions and all age groups. After learning of the emergence of SARS-CoV-2 virus in December 2019, and its potential for global dissemination to cause COVID-19 disease was realized, there was an urgent need to develop vaccines at an unprecedented rate and scale. As we appreciate and quantify the health, economic and social benefits of vaccines and immunization programs to individuals and society, we should endeavor to communicate this to the public and policy makers, for the benefit of endemic, epidemic, and pandemic diseases.
Introduction
“The impact of vaccination on the health of the world’s peoples is hard to exaggerate. With the exception of safe water, no other modality has had such a major effect on mortality reduction and population growth” ( Plotkin and Mortimer, 1988 ).
The development of safe and efficacious vaccination against diseases that cause substantial morbidity and mortality has been one of the foremost scientific advances of the 21st century. Vaccination, along with sanitation and clean drinking water, are public health interventions that are undeniably responsible for improved health outcomes globally. It is estimated that vaccines have prevented 6 million deaths from vaccine-preventable diseases annually ( Ehreth, 2003 ). By 2055, the earth’s population is estimated to reach almost 10 billion ( United Nations Department of Economic and Social Affairs, 2019 ), a feat that in part is due to effective vaccines that prevent disease and prolong life expectancy across all continents. That said, there is still much to be done to ensure the financing, provision, distribution, and administration of vaccines to all populations, in particular those which are difficult to reach, including those skeptical about their protective value and those living in civil disruption. Agencies including the World Health Organization (WHO), United Nations Children’s Fund (UNICEF), Gavi, the Vaccine Alliance, The Bill & Melinda Gates Foundation, and the Coalition for Epidemic Preparedness Initiative (CEPI), with their multiple funding streams have been instrumental in expanding vaccine benefits to all. These importance of these organizations in global co-operation and participation was essential in the setting of the 2019 global pandemic of SARS-CoV-2, in light of the health and economic impact of COVID-19 on societies in high-, middle- and low-income countries. This review will highlight the benefits of vaccinations to society from the perspectives of health, economy, and social fabric ( Figure 1 ), which need to be considered in the overall assessment of impact to ensure that vaccines are prioritized by those making funding decisions.

The impact of vaccines according to their health, economic or social benefit.
Brief History of Vaccine Development
Human use of preparations to prevent specific infections have been described since 1500 AD, beginning in China ( Needham, 2000 ) where smallpox was prevented by variolation, which is the introduction of material from scabs into the skin. In 1796 in the United Kingdom, Edward Jenner observed the immunity to smallpox of milkmaids having previously had natural infection with cowpox ( Jenner, 1798 ). He determined that inoculating small amounts of pus from the lesions of cowpox, presumably containing a virus related to vaccinia, into susceptible hosts rendered them immune to smallpox. The vaccine against smallpox was developed in 1798. The next phase of scientific developments involving the manipulation of infectious agents to extract suitable vaccine antigens took almost a century of research. Louis Pasteur’s work with attenuation by oxygen or heat led to live-attenuated chicken cholera, inactivated anthrax and live-attenuated rabies vaccines at the turn of the 20th century ( Pasteur, 1880 , 1881 , 1885 ). Alternative methods of attenuation using serial passage of Mycobacterium bovis led to the live Bacille Calmette-Guerin (BCG) ( Calmette, 1927 ) vaccine, still in use today for the prevention of tuberculosis. Serial passage was also used in the development of yellow fever vaccines ( Theiler and Smith, 1937a ) which are grown in chicken embryo tissues ( Theiler and Smith, 1937b ). Whole cell killed bacterial vaccines were developed when methods to treat and kill bacteria through heat or chemicals were established and whole cell typhoid, cholera and pertussis vaccines resulted at the end of the 19th Century. In 1923, Alexander Glenny and Barbara Hopkins developed methods to inactivate bacterial toxins with formaldehyde, leading to the diphtheria and tetanus toxoid vaccines ( Glenny and Hopkins, 1923 ).
Advances in virus culture in vitro allowed viral pathogens to be studied in greater detail and attenuation methods due to cultivation in artificial conditions led to the live oral polio, measles, rubella, mumps and varicella virus vaccines. In the 1960’s at the Walter Reed Army Institute of Research, vaccines were developed using capsular polysaccharides ( Gold and Artenstein, 1971 ; Artenstein, 1975 ), of encapsulated organisms including meningococci and later pneumococci ( Austrian, 1989 ) and Haemophilus influenzae type b (Hib) ( Anderson et al., 1972 ). To protect against multiple serotype variants of polysaccharide capsules, polyvalent vaccines were developed and later conjugated to carrier proteins to enhance their efficacy in infants in particular by recruiting T-cell mediated help to induce memory B-cells ( Schneerson et al., 1980 ). Vaccines made solely from proteins were rare, with the exception of the toxoid vaccines, but the acellular pertussis vaccine containing five protein antigens, was developed to mitigate the unwanted effects of the whole cell vaccine ( Sato and Sato, 1999 ).
The end of the 20th century marked a revolution in molecular biology and provided insights into microbiology and immunology allowing a greater understanding of pathogen epitopes and host responses to vaccination. Molecular genetics and genome sequencing has enabled the development of vaccines against RNA viruses possessing multiple variants of epitopes, such as the live and inactivated influenza vaccines ( Maassab and DeBorde, 1985 ) and live rotavirus vaccines ( Clark et al., 2006 ). DNA manipulation and excision allowed the use of surface antigen for hepatitis B viral vectors ( Plotkin, 2014 ). The human papilloma virus (HPV) vaccine benefits from enhanced immunogenicity due to the formation of virus-like particles by the L1 antigen of each virus contained in the vaccine ( Kirnbauer et al., 1992 ). Bacterial genome sequencing has provided in depth analysis of meningococcal antigens, to identify potential proteins for meningococcal B vaccines ( Serruto et al., 2012 ).
Vaccine development was tested in 2020 when a novel coronavirus, SARS-CoV-2, emerged from China causing a severe acute respiratory illness, which subsequently spread globally. Within 5 months of the discovery of this virus (7th January 2020) ( Zhu et al., 2020 ) and person-person transmission ( Chan et al., 2020 ), 5,697,334 cases had been identified, with orders of magnitude likely not measured and almost no country escaped the pandemic. Owing to the previous advances in vaccinology, by 8th April 2020, there were 73 vaccine candidates under pre-clinical investigation ( Thanh Le et al., 2020 ). Of these, six were in Phase 1 or 1/2 trials and one was in Phase 2/3 trials by 28th May 2020. The rapidity of this response demonstrated the ability to harness existing technologies including: RNA vaccine platforms ( {"type":"clinical-trial","attrs":{"text":"NCT04283461","term_id":"NCT04283461"}} NCT04283461 ), DNA vaccine platforms ( {"type":"clinical-trial","attrs":{"text":"NCT04336410","term_id":"NCT04336410"}} NCT04336410 ), recombinant vector vaccines ( {"type":"clinical-trial","attrs":{"text":"NCT04313127","term_id":"NCT04313127"}} NCT04313127 , {"type":"clinical-trial","attrs":{"text":"NCT04324606","term_id":"NCT04324606"}} NCT04324606 ) and adjuvants. The regulation, manufacturer and distribution of these vaccines will require expedition given the global public health need, from a period of many years to a matter of months. The efficacy and health impact of these vaccines is yet to be established, but if they are effective, then vaccines need to be made available for all global regions affected by SARS-CoV-2. The funding of this endeavor will prove challenging in a global context of national social and economic lockdown and massive government borrowing, but the justification for this provision will be through the multiple benefits to society that will need healthy citizens to rebuild economies in the decades post-COVID-19.
The history of vaccination is not complete without describing the public health intervention that led to the routine use of these vaccines for children globally. The Expanded Program of Immunization (EPI) was founded by WHO in 1974 with the aim of providing routine vaccines to all children by 1990 ( World Health Assembly, 1974 ). In 1977, global policies for immunization against diphtheria, pertussis, tetanus, measles, polio, and tuberculosis were set out. The EPI includes hepatitis B, Hib, and pneumococcal vaccines in many areas and by 2017, 85% of the world’s children (12–23 months of age) received diphtheria, pertussis, tetanus, and measles vaccines ( World Bank, 2019 ).
Health Benefits of Vaccination
Reduction in infectious diseases morbidity and mortality.
The most significant impact of vaccines has been to prevent morbidity and mortality from serious infections that disproportionately affect children. Vaccines are estimated to prevent almost six million deaths/year and to save 386 million life years and 96 million disability-adjusted life years (DALYs) globally ( Ehreth, 2003 ). The traditional measures of vaccine impact include: vaccine efficacy, the direct protection offered to a vaccinated group under optimal conditions e.g., trial settings; or vaccine effectiveness, the direct and indirect effect of vaccines on the population in a real-life setting ( Wilder-Smith et al., 2017 ). Providing a numerical measure of vaccine impact therefore involves estimating the extent of morbidity and mortality prevented. In the United States in 2009, amongst an annual birth cohort vaccinated against 13 diseases it was estimated that nearly 20 million cases of disease and ∼42,000 deaths were prevented ( Zhou et al., 2009 ). Infectious diseases that accounted for major mortality and morbidity in the early 20th century in the United States all showed over a 90% decline in incidence by 2017 from the pre-vaccine peak incidence ( Roush and Murphy, 2007 ), due to high vaccine uptake of over 90% for the DTaP (diphtheria, tetanus, and acellular pertussis), MMR (measles, mumps, and rubella) and polio vaccines ( World Health Organisation, 2019a ; Table 1 ). A similar pattern of infectious diseases reduction was seen across other high-income countries, demonstrating the efficacy of vaccines when available and accessible.
Vaccine impact in United States comparing the incidence of diseases prior to the implementation of vaccine ( Roush and Murphy, 2007 ), described as the pre-vaccine era and the vaccine coverage ( Hill et al., 2017 ) and disease incidence ( Centers for Disease Control and Prevention, 2017 ) in 2017, as reported by the Centers for Disease Control and Prevention.
Globally, the provision of vaccines is more challenging in many low- and middle- income countries (LMIC), as evidenced by the failure to make the EPI vaccines available to every child by 1990, irrespective of setting ( Keja et al., 1988 ). Central to this is limited financial resources, but other barriers to vaccine introduction include: underappreciation of the value of vaccines locally/regionally though insufficient relevant data on disease burden, vaccine efficacy, or cost-effectiveness; inadequate healthcare infrastructure for vaccine handling, storage, programmatic management, and disease surveillance; and lack of global, regional or local policy-making and leadership ( Munira and Fritzen, 2007 ; Hajjeh, 2011 ). In 2018, the global uptake of three doses of DTaP reached 86% which corresponded to 116,300,000 infants ( World Health Organisation, 2019a ). The vaccine coverage is, however, variable between low-, middle- and high-income countries because of a combination of economic and political circumstances as well as variable access to non-governmental support from Gavi, the Vaccine Alliance ( Turner et al., 2018 ; Figure 2 ). Nevertheless, there has been a decrease in the global burden of diseases caused by vaccine-preventable pathogens ( Figure 3 ) enabling healthier lives for many millions of children. A further benefit following vaccination, is the evidence that although vaccines may not always prevent an infection, for example VZV or pertussis, a milder disease course may follow ( Andre et al., 2008 ; Bonanni et al., 2015 ).

Vaccine uptake across different regions defined by economic status by the World Bank into high- (solid line), middle- (dashed line), and low-income countries (dotted line) for the past 20 years. Data from the World Health Organization and UNICEF dataset “Coverage Estimates Series” ( World Health Organization [WHO] and United Nations Children’s Fund [UNICEF], 2019 ).

Reduction in infectious diseases globally. Across all world regions, data from the WHO, for the last 20 years showing the control of diphtheria and tetanus and the decline in rubella and congenital rubella syndrome (data not shown). Data from the World Health Organization dataset “Reported cases of vaccine-preventable diseases” ( World Health Organisation, 2019c ).
Eradication of Infectious Diseases
Global disease eradication can be achieved for pathogens that are restricted to human reservoirs. For eradication of infectious diseases, high levels of population immunity are required globally, to ensure no ongoing transmission in our well-connected world ( Andre et al., 2008 ). Furthermore, surveillance systems must be in place to monitor the decline in disease, with accurate and reliable diagnostic testing to monitor ongoing cases. At the time of writing, the only infectious disease that has been eradicated in humans by vaccination is smallpox. This disease had afflicted humans for millenia, with the earliest evidence found in Egyptian mummies from 1000 BC ( Geddes, 2006 ). Jenner’s successful development of the smallpox vaccine using vaccinia virus ( Jenner, 1798 ) led to the ultimate eradication of the disease through ring vaccination as announced by the World Health Assembly in 1980 ( Strassburg, 1982 ), which was an historic public health achievement. The second example of eradication was of the rinderpest virus in livestock, an infection that indirectly led to human loss of life through loss of agriculture leading to humanitarian crises through famine and poverty. Rinderpest virus infects cattle, buffalo and numerous other domestic species, with widespread disease affecting large parts of Africa and Europe in the 19th century ( Roeder et al., 2013 ). The Plowright tissue culture rinderpest vaccine, developed during the 1950s, was used for mass vaccination campaigns, alongside other public health measures, leading to eradication in 2011 ( Morens et al., 2011 ).
The next infection targeted for eradication is wild polio virus. This devastating paralytic disease routinely afflicted children and adults in both industrialized and developing settings, prior to the development of vaccines. Two polio vaccines, the inactivated polio vaccine (IPV) and the live-attenuated oral polio vaccine (OPV) became available in 1955 and 1963, respectively ( Plotkin, 2014 ), both able to protect against all three wild types of polio virus. Both vaccines have been used globally, with live-attenuated OPV much cheaper and easier to administer but carrying the risk of causing circulating vaccine-derived poliovirus (cVDPV) owing to back-mutation and re-acquisition of neurovirulence. Hence, due to its safety IPV was preferred in industrialized regions and those where the polio incidence was low. In 1998, the Global Polio Eradication Initiative, the largest public-private partnership led by national governments in partnership with the WHO, Rotary International, United States Centers for Disease Control and Prevention (CDC), and UNICEF was launched with the aim of global polio eradication by 2000. Although this target was not met due to lack of funding, political will, and competing health initiatives, there was a 99% reduction in polio incidence by 2000 ( Lien and Heymann, 2013 ). By 2003, there were only six endemic countries with new cases: Egypt, Niger, India, Nigeria, Afghanistan, and Pakistan, of which only the latter four had new cases by 2005. Eradication in India was problematic due to the high birth rates and poor sanitation amongst densely populated regions with marginalized communities and high population mobility ( Thacker et al., 2016 ). India was declared polio free in 2014. Wild polio virus type 2 was eradicated in 2015, the last case of wild type 3 was in 2012 and eradication announced in 2019, with wild type 1 virus remaining in two countries, Pakistan and Afghanistan ( World Health Organisation, 2019b ). In 2019, Nigeria was declared 3 years free of wild polio, the last country in Africa to declare any cases. In the first 6 months of 2020, there were 51 and 17 cases of wild type 1 polio reported in Pakistan and Afghanistan respectively ( Global Polio Eradication Initiative, 2019 ). Ongoing programs to roll out universal vaccination in both countries remain hindered by armed conflict, political instability, remote communities and underdeveloped infrastructure. The risk of the OPV recipients developing cVDPV disease, with transmission through the faeco-oral route to cause outbreaks of vaccine-derived paralytic poliomyelitis remains a concerning obstacle in the eradication process, requiring intensive surveillance.
Herd Immunity
The overriding health benefit perceived by most vaccine recipients is their personal, direct, protection. The added value of vaccination, on a population level, is the potential to generate herd immunity. Where a sufficiently high proportion of the population are vaccinated, transmission of the infecting agent is halted thereby protecting the unvaccinated, who may be those too young, too vulnerable, or too immunosuppressed to receive vaccines. Highly successful vaccination programs have been in place as part of the routine EPI, against encapsulated bacteria that are carried asymptomatically in the oropharynx but that can invade and cause septicemia and meningitis in all age groups. Vaccines against Neisseria meningitidis ( Gold and Artenstein, 1971 ), Streptococcus pneumoniae ( Austrian, 1989 ), and Hib ( Anderson et al., 1972 ) were developed in the 1960s, 1970s, and 1980s, respectively, using their polysaccharide capsules as vaccine antigens, which successfully induced protective immunity (direct protection). Conjugation of these polysaccharides to carrier proteins in the 1990s improved their efficacy by not only ensuring a T cell response and immune memory, but by reducing acquisition of pharyngeal carriage of these organisms, thus providing indirect protection and thereby preventing ongoing transmission ( Pollard et al., 2009 ). This was first observed in national carriage studies in the United Kingdom in 1999–2001 during a mass vaccination campaign against serogroup C N. meningitidis ( Maiden et al., 2008 ) and was a major contributing factor to the declining disease thereafter.
Herd (population) immunity requires high levels of vaccine uptake, to limit the number of unvaccinated people and the opportunity for pathogen transmission between them. The proportion of a given population required to induce herd immunity through vaccination is lower for the bacterial infections and conjugate polysaccharide vaccines, as their basic reproductive number (R 0 ) is lower than viral infections like measles, varicella or polio ( Table 2 ). Measles virus can cause devastating disease ranging from acute presentations with pneumonia or encephalitis, to immune amnesia and long-term complications such as subacute sclerosing panencephalitis ( Mina et al., 2015 , 2019 ; Moss, 2017 ; Petrova et al., 2019 ). The live-attenuated measles vaccine is highly efficacious and the first dose is recommended at 9–12 months of age. To protect those who cannot receive live vaccines (younger infants, pregnant women, the immunosuppressed) from acquiring measles in the community, at least 93–95% of the population is required to be vaccinated with two doses in order to interrupt measles virus transmission. In many countries in Europe and in the United States, this level of vaccination uptake is falling ( Wise, 2018 ), due to a combination of reduced accessibility to health services and vaccine misinformation. As a result, some countries, including the United Kingdom and United States, where elimination of measles had been declared have had a resurgence of disease ( Wise, 2019 ). For high-risk individuals who are unable to be vaccinated, herd immunity represents a life-saving protection strategy against many infections. An alternative strategy, cocooning, has been employed with limited success for pertussis and influenza ( Grizas et al., 2012 ), where their close/household contacts are vaccinated to prevent transmission.
Vaccines with the potential to induce herd immunity, with the infectious agent, vaccine type, and thresholds of population vaccination needed for herd immunity ( Peltola et al., 1999 ; Whitney et al., 2003 ; Donaghy et al., 2006 ; Fine and Griffiths, 2007 ; Maiden et al., 2008 ; Curns et al., 2010 ; Paulke-Korinek et al., 2011 ; Plans-Rubio, 2012 ; Daugla et al., 2014 ; Tabrizi et al., 2014 ; Funk et al., 2019 ; Palmer et al., 2019 ).
Herd immunity has been observed for gastrointestinal infections with vaccines against cholera (oral cholera vaccine) and rotavirus (oral rotavirus vaccines). Early adopters of rotavirus vaccines included the United States (2006) and Austria (2007) where there were dramatic reductions in disease observed in the vaccinated infant cohort, and also in the older age groups of children and adults ( Curns et al., 2010 ; Paulke-Korinek et al., 2011 ), suggesting that the reduction in disease and shedding of virus in the stool stopped transmission to healthy household contacts. For the OPV, herd protection may also be induced through vaccine virus shedding and spread to unvaccinated people ( Fine and Griffiths, 2007 ).
Reduction in Secondary Infections That Complicate Vaccine-Preventable Diseases
Vaccines can prevent diseases beyond the specific infection they are designed to target. Infections with pathogens, in particular viruses, can predispose to the acquisition of other bacterial infections. For example, influenza virus infection, both seasonal and pandemic, is frequently complicated by bacterial pneumonia and acute otitis media (OM), and infrequently Aspergillus pneumonia/pneumonitis. During the influenza pandemic of 1918–19, secondary bacterial bronchopneumonia with S. pneumoniae, Streptococcus pyogenes , H. influenzae , and Staphylococcus aureus identified at autopsy, likely contributed to the excess mortality observed amongst healthy children and adults ( Morens and Fauci, 2007 ). Influenza vaccinations can be beneficial in preventing these complications and also morbidity including acute OM in children; a systematic review demonstrated influenza vaccine efficacy against OM of 51% (21–70%) ( Manzoli et al., 2007 ). Further, there is evidence that inactivated influenza vaccines administered to pregnant women can reduce the hospital admission with acute respiratory illnesses in their infants up to 6 months of age ( Regan et al., 2016 ). Amongst pregnant, HIV-negative women in South Africa, infants (<3 months) were protected against hospitalization with all-cause lower respiratory tract infections with a vaccine efficacy of 43% ( p = 0.05), including primary viral and secondary bacterial causes ( Nunes et al., 2017 ). Additionally, in children pneumococcal conjugate vaccines were observed to reduce the incidence of influenza-associated hospital admissions in United States ( Simonsen et al., 2011 ), Spain ( Dominguez et al., 2013 ), and South Africa ( Madhi et al., 2004 ; Abadom et al., 2016 ), through the prevention of secondary bacterial infections following primary influenza infection.
The introduction of the live-attenuated measles vaccine in the 1970s was observed to reduce both measles and non-measles mortality in children ( Aaby et al., 2003 ). Measles causes severe pneumonia, encephalitis, and the long-term sequel of subacute sclerosing panencephalitis ( Moss, 2017 ), but the decline in mortality was not limited to preventing these alone ( Aaby et al., 2003 ). Mathematical modeling of vaccination and immunological research demonstrated that measles causes an immunological amnesia, eliminating B cell populations and thus immune memory, leaving measles survivors susceptible to all the infective agents they had previously developed immunity against; it is estimated to take 3 years for immune recovery to occur ( Mina et al., 2015 ).
Prevention of Cancer
Historically, vaccines were developed against very severe infections with major morbidity and mortality from acute disease. As non-communicable diseases, including cancer, become the most frequent causes of death in industrialized countries and some developing countries, vaccines are being used to prevent these too, when the infectious agents are involved in carcinogenesis. Hepatitis B prevalence is high in regions of East Asia, sub-Saharan Africa, and the Pacific Islands. Chronic hepatitis B infection can lead to liver cirrhosis and hepatocellular carcinoma ( Bogler et al., 2018 ). Vertical transmission of hepatitis B is problematic as 70–90% of babies born to HbsAg and HbeAg positive mothers will become infected without prophylaxis administered to babies; with ∼90% of infants developing chronic hepatitis ( Borgia et al., 2012 ; Gentile and Borgia, 2014 ). The chronic hepatitis B carriage status of mothers is routinely checked at the start of pregnancy, in order to assess the need to vaccinate the infant after birth. The use of both hepatitis B vaccine, containing hepatitis B surface antigen, and immunoglobulin containing hepatitis B antibody can be used to minimize vertical transmission, with evidence from a 20-year-long study in Thailand demonstrating 100% prevention of transmission ( Poovorawan et al., 2011 ).
The sexually transmitted HPV is responsible for genital tract and oropharyngeal infections as a precursor to causing oncological disease affecting the cervix, vagina, vulva, penis, anal tract, and pharynx in both men and women. Cervical cancer is the fourth most common cancer globally, with 528,000 new cases annually and peak incidence in young women aged 25–34 years ( Ferlay et al., 2012 ). The HPV serotypes 16 and 18 carry a high-risk for cervical cancer ( Wang et al., 2018 ) and vaccination against these specific serotypes has been available since 2006 through bivalent (16 and 18), quadrivalent (6, 11, 16, and 18), and nonavalent (6, 11, 16, 18, 31, 33, 45, 52, 58) vaccines, which are now available to individuals from the age of 9 years ( Gupta et al., 2017 ). A vaccination program started in the United Kingdom in 2008, and at the time of writing over 10.5 million doses had been given to girls ( Public Health England, 2018 ), with the aim of preventing primary infection with HPV. The vaccine coverage was 83.8% for 13–14 year old girls in England in 2017/18 ( Public Health England, 2019 ). In July 2018, the vaccine was approved for use in boys ( Public Health England, 2019 ). After a decade of use, there has been an observed decline in the genital infections caused by serotypes 16 and 18 ( Public Health England, 2018 ), with further time needed to observe the fall in cervical cancer incidence. However, the incidence of pre-invasive cervical diseases has been reduced by 79–89% in Scottish women over 20 who were vaccinated with bivalent HPV vaccine when aged 12–13 years, with evidence of herd protection ( Palmer et al., 2019 ), offering a promising outlook for the reduction of cervical cancer in the future. An additional benefit of HPV vaccines, is their impact on neonatal morbidity and mortality, through the reduction in surgical treatment of cervical neoplasias, and the related preterm births and complications ( Soergel et al., 2012 ).
Preventing Antibiotic Resistance
The rise in antimicrobial resistance (AMR) is a universal threat. The use of antibiotics in humans, exposes the bacteria that reside in our microbiota to selection pressures resulting in the development of AMR. As the bacteria constituting the host microbiota are frequently responsible for invasive diseases such as: meningitis, pneumonia, urinary tract, or abdominal infections, the risk of developing infections that are difficult or eventually impossible to treat is fast becoming a reality ( Brinkac et al., 2017 ). In regions where resistant pathogens are circulating at high frequency, such as India or regions of Europe ( Logan and Weinstein, 2017 ), patients will be faced with choosing between having elective surgical procedures or chemotherapy for malignancy, and the risk of acquiring potentially untreatable, multi-drug resistant bacterial infections ( Liu et al., 2016 ). Vaccination is crucial in mitigating this risk, by preventing people from developing viral and bacterial infections in the first instance, and therefore reducing the antibiotic burden to which their microbiota are exposed. The development of AMR in bacteria is a cumulative process with frequent, repeated exposure to broad spectrum antibiotics as a major driver. Children and the elderly who are at particular risk of infection can benefit from vaccines against common primary and secondary infections such as: pneumonia (prevented by PCV, PPSV, influenza, and measles vaccines), OM (PCV, Hib, and measles vaccines), cellulitis secondary to VZV (VZV vaccine), and typhoid fever (typhoid vaccine) which alleviates the need for antibiotics being prescribed or bought ( Kyaw et al., 2006 ; Palmu et al., 2014 ). The extent to which vaccination contributes to antimicrobial stewardship was highlighted by its inclusion in vaccine cost-effectiveness analyses as part of national United Kingdom policy ( Bonanni et al., 2015 ).
Economic Benefits
Cost savings.
Vaccines are highly beneficial on a population level and also cost-effective ( Shearley, 1999 ) in comparison to other public health interventions ( Bloom et al., 2005 ). Government departments are required to perform systematic economic analyses of vaccines and vaccine programs to justify their purchase in view of pressure on public and private finances globally, this was exacerbated by the 2008 financial crash. A vaccination program has clear direct costs including: vaccine purchase, infrastructure to run the program and maintain the cold chain, and healthcare/administration personnel. Governments, sometimes supported by charities and non-governmental organizations, invest in these with the intention of improving health. The reduction in morbidity and mortality associated with successful vaccine programs, through a combination of direct and indirect protection, has led to reduced incidence of diseases and their associated treatments and healthcare costs ( Deogaonkar et al., 2012 ). This potentially leads to economic growth, with less money spent owing to the costs averted through fewer medical tests, procedures, treatments and less time off work by patients/parents. Additionally, the use of combination vaccines e.g., DTaP/IPV/Hib/HepB provides protection against an increased number of diseases, with no additional infrastructure costs i.e. the same number of injections per child within existing immunization programs.
The cost-effectiveness analyses of vaccination programs demonstrate that they are overwhelmingly worth the investment, with most programs costing less than $50 per life gained, orders of magnitude less than prevention of diseases like hypertension ( Ehreth, 2003 ; Bloom et al., 2005 ). The returns on investment in vaccines, given their increasing provision through Gavi, have been estimated at 12–18% ( Bloom et al., 2005 ), but this is likely an underestimate. The monetary advantages of vaccination programs are important both to industrialized nations, such as the United States which obtains a net economic benefit of $69 billion, but also in 94 LMIC where investment of $34 billion, resulted in savings of $586 billion from the direct illness costs ( Ozawa et al., 2016 ; Orenstein and Ahmed, 2017 ). The net economic impact of eradication of disease has been estimated for both smallpox and polio. For smallpox, the eradication costs were over 100 million USD, but there are cost savings of 1.35 billion USD annually, with elimination of polio estimated to save 1.5 billion USD annually ( Barrett, 2004 ; Bloom et al., 2005 ). A less well-considered economic saving, not captured in cost-effectiveness or cost-benefit analyses, is from the prevention of long-term morbidity following acute infections ( Bloom et al., 2005 ), for example hearing impairment following pneumococcal meningitis or limb amputation following meningococcal disease, along with broader productivity gains ( Deogaonkar et al., 2012 ), which could have a major impact on LMIC adoption of vaccine programs.
Productivity Gains
The relationship between health and the economy is bidirectional, whereby economic growth enables funding in investments that improve health; and a healthy population contributes to and enhances an economy. These benefits of vaccinations and other public health interventions including sanitation, clean water, and antibiotics, are important for social as well as economic reasons. It has been suggested that the economic impact of vaccines should be considered more broadly than just the averted healthcare costs from prevented illness episodes and associated carer costs ( Deogaonkar et al., 2012 ; Barnighausen et al., 2014 ; Bonanni et al., 2015 ; Gessner et al., 2017 ; Wilder-Smith et al., 2017 ). Bärnighausen et al. (2011) , set out a framework to consider productivity gains measured by: outcome and behavior; community health and economic externalities; risk reduction; and health gains. Healthy children demonstrate improved educational attainment at school through better attendance and better cognitive performance ( Barham and Calimeria, 2008 ; Bloom et al., 2011 ; Deogaonkar et al., 2012 ). The impact of hearing loss from mumps, rubella or pneumococcal infections, or visual impairment from measles may require specific educational support, whereas the cognitive deficits from those childhood infections may require substantial remedial input. As more children survive to adulthood, a larger adult workforce is available, who when healthy can work for longer and more productively both physically and mentally ( Bloom and Canning, 2000 ; Bloom et al., 2005 ); though to date this has been observed largely following other health improvements, not vaccination specifically ( Jit et al., 2015 ). As a result of vaccination healthy and economically successful populations have lower fertility rates and smaller families ( Sah, 1991 ; Andre et al., 2008 ). With improved health and therefore life expectancy, there is a wider effect on families who may choose to invest more money in their future, for example to enhance their education or through savings ( Jit et al., 2015 ). Overall, vaccine programs should be viewed as an investment in human capital, providing enduring impact on economies worldwide.
Minimizing the Impact on Family
The economic impact of adult illness is evident from loss of productivity and pay for the duration of the illness and recovery period. The impact of childhood illness falls primarily on their adult carers, generally parents. In most industrialized regions, two-parent families are reliant on both parents undertaking at least part-time or full-time work. Therefore, when a child is unwell with childhood illnesses, which may or may not necessitate admission to hospital, the parent will invariably have to forego their paid employment to care for the child. In seven European countries one parent or carer required time off work in 39–91% of rotavirus gastroenteritis cases ( Van der Wielen et al., 2010 ). This loss of productivity in the parental workforce tends to disproportionately affect women, but loss of either parental attendance at work reduces overall employer productivity and in the short-term is rarely replaced. This argument was made for the impact of chicken pox on children, whereby the exclusion from school mandates parental caring at home for a period until the lesions are crusted over. VZV vaccines are estimated to have had a similar impact as rotavirus vaccine in United States studies ( Lieu et al., 1994 ). In many regions, mothers are still the primary carers, spending their days at home caring for children and maintaining the household; in these settings, the impact on this unpaid work is harder to determine.
It is of paramount importance to quantify and include productivity gains and the wider effects in analyses of impact for vaccines with only moderate efficacy, as calculated using traditional metrics. Vaccines such as the RTS,S/AS01 malaria vaccine, CYD-TDV dengue vaccine and rotavirus vaccine used in LMIC all have limited ability to broadly protect populations over a long duration but the public health benefits were important in vaccine implementation decisions in those countries ( Wilder-Smith et al., 2017 ). This suggests a paradigm for alternative regulatory requirements with a focus on public health outcomes ( Gessner et al., 2017 ).
Cost-Effective Preparedness for Outbreaks
As human populations grow and their use of the finite land resources increases, we are in increasingly close association with other living creatures, voluntarily or involuntarily. This interaction with natural reservoirs of potential infectious diseases increases the risk of zoonotic transmission of new infectious pathogens e.g., SARS, MERS-CoV, or known infectious pathogens with increased virulence e.g., influenza. Emerging infectious diseases disproportionately affect developing regions, where health infrastructure and surveillance are likely to be less well-established and robust. There were 1,307 epidemics of infectious diseases between 2011 and 2017, which cumulatively cost $60 billion annually to manage ( GHRF Commission, 2016 ). The unpredictability of outbreaks was highlighted by the Ebola epidemic in Western African countries of Liberia, Sierra Leone, and Guinea in 2014, which occurred in a period when public health was supposedly at its most advanced in recent history. However, a catalog of areas including: outbreak planning infrastructure; disease surveillance; local health services; escalation to international agencies were found to be lacking ( GHRF Commission, 2016 ). Although the WHO received criticism for its lack of escalation, in reality the global and interconnected infrastructure to prevent such epidemics taking lives and devastating societies is insufficient at the present time. The Zika virus epidemic in Latin America in 2015, first observed through an unexpectedly high incidence of microcephaly amongst newborns in Brazil’s northern regions ( Heukelbach et al., 2016 ), provide another example of how epidemics can have lasting impact, with the virus causing significant neurological damage to surviving infants ( Russo et al., 2017 ). The SARS-CoV-2 pandemic which began in 2019, was, at the time of writing, the largest infectious disease pandemic since the influenza pandemic of 1918/9. This global public health crisis highlighted stark societal inequalities persistent in many high-, middle- and low-income countries with direct and indirect impact on health outcomes from this infection. The cost-effectiveness of a vaccine in this setting was unquestionable, with economies and societies shut down for months in early 2020 and likely again in future. As it is not feasible or practical to be able to predict the location or nature of the next emerging threat, investment of an estimated $4.5 billion/year in healthcare systems could help speed up responses to infectious epidemics by prompt identification of the agent and effective control measures to limit the spread and consequences of disease ( GHRF Commission, 2016 ). The importance of this planning within the political landscape and the ongoing threat that infectious disease pose, may be appreciated more widely after 2020.
Establishing Programs for Vaccine Development
One effective infection control method is the use of vaccines in the course of an epidemic to halt transmission and to induce immunity to those as yet unaffected. The cost of vaccine development is a major challenge as there is little incentive for industry to invest in the design, testing and manufacture of vaccines that may never be needed, have a limited market, and, as previously eluded to, may be required in LMIC which cannot afford the upfront costs as an epidemic unfolds. The estimated costs for funding the development of infectious diseases vaccines for epidemics through phase 2a clinical trials are a minimum of $2.8-3.7 billion ( Gouglas et al., 2018 ). The CEPI alliance was established at the Davos World Economic Forum in 2017 as a global partnership between public, private and philanthropic organizations. In response to the conclusion that “a coordinated, international, and intergovernmental plan was needed to develop and deploy new vaccines to prevent future epidemics,” CEPI have identified the most important known global infectious threats and invested in the development of vaccines, stockpiling, and policies to allow equitable access to these ( Plotkin, 2017 ). Further, the establishment of research and development infrastructure pipelines will allow production of suitable vaccine candidates within 16 weeks of identification of a new pathogen antigen. The broader aims including: improving global epidemic responses; capacity building; and global regulation of outbreak management strategies are also within the remit of CEPI’s work. It is these types of preparedness plans that assisted vaccine development and global health collaborations to address the COVID-19 pandemic, though many regions of high-, middle-, and low-income countries alike were slow or resistant to pre-empt and prepare for this type of infectious disease threat.
Social Benefits
Equity of healthcare.
As a result of the combined effects of poverty, malnutrition, poor hygiene and sanitation, overcrowding, discrimination and poorer access to health-care, the underprivileged in society are disproportionately afflicted by infectious diseases. Over the 20th century, it has become a moral standpoint and a human right for every individual to be provided with access to safe vaccines. The provision of vaccination as part of the EPI on a national and international scale ( World Health Assembly, 1974 ) acted as a great leveler to start reducing the impact of infectious diseases to all, regardless of other disadvantages. Over the 15 years of the EPI, the vaccine coverage in developing countries increased from 5% to ∼80% ( Levine and Robins-Browne, 2009 ). The EPI was revolutionary for its time, an ambitious public health program that aimed to improve children’s life chances despite the country and situation in which they were born. The administration of vaccines by UNICEF was deemed so important that there have been at least seven ceasefires in civil conflicts to allow this to happen ( Hotez, 2001 ).
The impact of vaccines on the inequity of those living in poverty is marked. A study of over 16,000 children during the phased introduction of the measles vaccine in Bangladesh in 1982, demonstrated improved health outcome equity when measured by under-5 mortality ( Bishai et al., 2003 ). Further, modeling of the impact of the rotavirus vaccine in India across social strata, which are closely aligned to wealth, suggested that the vaccine program would provide the poor with both health and financial benefits ( Verguet et al., 2013 ). Including such equity impact in the health economic modeling of vaccines would allow policy decisions to be targeted to the most vulnerable in society ( Riumallo-Herl et al., 2018 ). Additional cost-effective benefits observed after the implementation of combined public health initiatives ( Deogaonkar et al., 2012 ; Gessner et al., 2017 ) include provision of vaccines, facilitation of healthcare, reduction of indoor air pollution and improvement of nutrition to prevent childhood pneumonia ( Niessen et al., 2009 ).
Strengthening Health and Social Care Infrastructure
To provide the EPI universally to infants and children, a significant degree of healthcare infrastructure is required ranging from primary care to public health. An example of the multiple facets of a successful vaccine program were outlined in the Mission Indradhanush in India, which planned to make life-saving vaccines available to all children and pregnant women by 2020 through programs with (i) national, (ii) state, (iii) district, and (iv) block/urban level input ( Hinman and McKinlay, 2015 ). National programs require governments to provide financial resources and set out policy for implementation. States needed to obtain the vaccines and to store them appropriately whilst eligible children were identified through public health messaging and outreach. Districts and urban areas recruited staff trained in vaccine delivery and communication to administer vaccines and to provide the aftercare where required. Establishing this degree of nationwide infrastructure to reach those in urban and rural areas, provides the basis for the provision of other health and social care services for all members of the community, in particular improving maternal and infant mortality in developing regions and in the elderly in industrialized regions ( Shearley, 1999 ). Public health infrastructure and personnel could be used to promote other important messages and health education ( Shearley, 1999 ), relating to malnutrition, hygiene and sanitation and preventable diseases such as malaria and HIV infection. Global drivers are also key, as demonstrated by the establishment of the EPI in 1974, when all countries were directed to provide these vaccines, thereby developing their primary- and public health-care infrastructure, with benefit beyond the vaccine program. Vaccination contributes to the UN Millennium Development Goals and later Sustainable Development Goals for achievement by 2030. Gavi, the Vaccine Alliance, has been an important provider of funds, vaccines and support for countries whose gross national income per capita was <£1000/year ( Hinman and McKinlay, 2015 ). The partnerships forged through the development of vaccine programs in LMIC, can be long-lasting and beneficial through other health and social care endeavors ( Shearley, 1999 ).
Impact of Life Expectancy and Opportunity
Vaccination programs provide a degree of social mobility, as poverty and the associated ill-health and mortality from infectious diseases are no longer the determinants of one’s life chances. Vaccine recipients have the potential for improved life-expectancy largely demonstrated by, but not confined to, infants and children ( Andre et al., 2008 ). It has become increasingly recognized that an aging population goes through the process of immunosenescence ( Fulop et al., 2017 ), and increased incidence and severity of infectious diseases. In many countries, therefore, older people are offered vaccines to prevent infections with high mortality and morbidity, including the influenza, pneumococcal, herpes zoster, and pertussis vaccines ( Bonanni et al., 2015 ). These prevent the development of pneumonia, admission to hospital and the subsequent associated risks of death from cardiac failure, as observed in Sweden ( Christenson et al., 2004 ).
The global and interconnected world of the 21st century provides opportunity to discover new cultures, new environments and their resident microbes. The safety of global travel has been greatly enhanced by the availability of vaccines that provide protection against organisms that are different to those in a person’s home setting. Movement of people may be through necessity when fleeing war and conflict, in the search of better life opportunities, or for leisure purposes. For mass movements of refugees vaccines are crucial to the aid and relief efforts to support these individuals ( Hermans et al., 2017 ), as measles and cholera can be highly problematic in refugee camps. Global mass cultural or religious gatherings, such as the Hajj pilgrimage ( Yezli et al., 2018 ) or the Chinese New Year ( Chen et al., 2018 ) have been implicated in the spread of meningococcal disease outbreaks. Pre-travel vaccines offer the optimal level of protection for those with scheduled travel plans and include protection against: yellow fever, hepatitis A and B, rabies, Japanese encephalitis, tick-borne encephalitis, typhoid, and cholera.
Empowerment of Women
The empowerment of women is both a driver and effect of vaccination programs. The degree of education, literacy and independence of girls and women varies considerably across the world and within countries. Where women have the information and autonomy to make health-related decision for their children, childhood immunization rates improve. In a study in Bihar State in rural India involving an empowerment program, where participating women were educated about health and hygiene, there was a higher rate of DTP, measles and BCG vaccination in their children compared to the non-participants in the villages running the program ( Janssens, 2011 ). Further, this information and autonomy served to improve the rates of vaccination in children of non-participants in the villages running the program compared to control villages not running the education program, through social or formal ongoing dialogue within the village community. A separate public health initiative in Haryana, India conducted between 2005 and 2012 to reduce maternal and child health inequalities, involved improving access and provision of health resources to rural areas, the poor in society, women and children. One significant outcome of this initiative was the equitable provision of immunizations to girls and boys, despite the male-favored disparity prior to starting the public health initiative ( Gupta et al., 2016 ).
By improving infant and childhood mortality from infection, more children will survive to adulthood with the potential to have productive and healthy lives. This has led to healthy and economically secure women having fewer children and less peripartum morbidity and mortality ( Sah, 1991 ; Shearley, 1999 ). Thus, women are able to spend more time with their children and on their development ( Shearley, 1999 ) as well as their own education and contribution to the workforce. The strategy of maternal vaccination has demonstrated great success at preventing diseases that afflict infants too young to be vaccinated against pertussis, influenza and tetanus ( Marchant et al., 2017 ). Factors influencing the uptake of maternal vaccination include women’s previous experiences with healthcare and vaccines, so it is crucial to provide the access and support required to enable them to make informed choices during their pregnancy ( Wilson et al., 2019 ).
The impact of vaccines is broad and far-reaching, though not consistently quantifiable, analyzed or communicated. Traditionally, the perceived benefits of vaccination were to reduce morbidity and mortality from infections, and those remain the drivers for the innovation of new vaccines, in particular in preparation for outbreaks or against infections that afflict the most disadvantaged in society. However, an increasing appreciation for the economic and social effects of vaccines is being included in the development and assessment of vaccine programs, potentially realizing a greater benefit to society and resulting in wider implementation. There remain challenges to delivering vaccines to all children and vulnerable people worldwide, in particular those in communities that are difficult to reach geographically, politically and culturally and these challenges can only be overcome with the continued commitment and dedication to this endeavor on an international, national and individual scale.
Author Contributions
SP conceptualized and designed the study. CR prepared the manuscript and figures. CR and SP contributed to literature search and revision and review of the final manuscript. Both authors contributed to the article and approved the submitted version.
Conflict of Interest
SP consults for many major vaccine manufacturers and biotechnology companies but this article was unfunded.
The remaining author declares that the research was conducted in the absence of any commercial or financial relationships that could be construed as a potential conflict of interest.
- Aaby P., Bhuiya A., Nahar L., Knudsen K., de Francisco A., Strong M. (2003). The survival benefit of measles immunization may not be explained entirely by the prevention of measles disease: a community study from rural Bangladesh. Intern. J. Epidemiol. 32 106–116. [ PubMed ] [ Google Scholar ]
- Abadom T. R., Smith A. D., Tempia S., Madhi S. A., Cohen C., Cohen A. L. (2016). Risk factors associated with hospitalisation for influenza-associated severe acute respiratory illness in South Africa: a case-population study. Vaccine 34 5649–5655. [ PMC free article ] [ PubMed ] [ Google Scholar ]
- Anderson P., Peter G., Johnston R. B., Jr., Wetterlow L. H., Smith D. H. (1972). Immunization of humans with polyribophosphate, the capsular antigen of Hemophilus influenzae , type b. J. Clin. Invest. 51 39–44. [ PMC free article ] [ PubMed ] [ Google Scholar ]
- Andre F. E., Booy R., Bock H. L., Clemens J., Datta S. K., John T. J., et al. (2008). Vaccination greatly reduces disease, disability, death and inequity worldwide. Bull. World Health Organ. 86 140–146. [ PMC free article ] [ PubMed ] [ Google Scholar ]
- Artenstein M. S. (1975). Control of meningococcal meningitis with meningococcal vaccines. Yale J. Biol. Med. 48 197–200. [ PMC free article ] [ PubMed ] [ Google Scholar ]
- Austrian R. (1989). Pneumococcal polysaccharide vaccines. Rev. Infect. Dis. 11 ( Suppl. 3 ), S598–S602. [ PubMed ] [ Google Scholar ]
- Barham T., Calimeria L. (2008). Long-term Effects of Family Planning and Child Health Interventions on Adolescent Cognition: Evidence from Matlab in Bangladesh. Boulder, CO: Univeristy of Colorado. [ Google Scholar ]
- Barnighausen T., Bloom D. E., Cafiero-Fonseca E. T., O’Brien J. C. (2014). Valuing vaccination. Proc. Nat. Acad. Sci. U.S.A. 111 12313–12319. [ PMC free article ] [ PubMed ] [ Google Scholar ]
- Bärnighausen T., Bloom D. E., Canning D., Friedman A., Levine O. S., O’Brien J., et al. (2011). Rethinking the benefits and costs of childhood vaccination: the example of the Haemophilus influenzae type b vaccine. Vaccine 29 2371–2380. [ PubMed ] [ Google Scholar ]
- Barrett S. (2004). Eradication versus control: the economics of global infectious disease policies. Bull. World Health Organ. 82 683–688. [ PMC free article ] [ PubMed ] [ Google Scholar ]
- Bishai D., Koenig M., Ali Khan M. (2003). Measles vaccination improves the equity of health outcomes: evidence from Bangladesh. Health Econ. 12 415–419. [ PubMed ] [ Google Scholar ]
- Bloom D. E., Canning D. (2000). Policy forum: public health. The health and wealth of nations. Science 287 : 1207 . [ PubMed ] [ Google Scholar ]
- Bloom D. E., Canning D., Seiguer E. (2011). The Effect of Vaccination on Children’s Physical and Cognitive Development in the Philippines. Boston, MA: Harvard School of Public Health. [ Google Scholar ]
- Bloom D. E., Canning D., Weston M. (2005). The value of vaccination. World Econ. 6 15–16. [ Google Scholar ]
- Bogler Y., Wong R. J., Gish R. G. (2018). “ Epidemiology and natural history of chronic hepatitis B virus infection ,” in Hepatitis B Virus and Liver Disease , eds Kao J.-H., Chen D.-S. (Berlin: Springer; ), 63–89. [ Google Scholar ]
- Bonanni P., Picazo J. J., Remy V. (2015). The intangible benefits of vaccination - what is the true economic value of vaccination? J. Mark. Access Health Policy 3 : 10.3402/jmahv3.26964 . [ PMC free article ] [ PubMed ] [ Google Scholar ]
- Borgia G., Carleo M. A., Gaeta G. B., Gentile I. (2012). Hepatitis B in pregnancy. World J. Gastroenterol. 18 4677–4683. [ PMC free article ] [ PubMed ] [ Google Scholar ]
- Brinkac L., Voorhies A., Gomez A., Nelson K. E. (2017). The threat of antimicrobial resistance on the human microbiome. Microb. Ecol. 74 1001–1008. [ PMC free article ] [ PubMed ] [ Google Scholar ]
- Calmette A. (1927). La Vaccination Preìventive Contre La Tuberculose par le “BCG,”. Paris: Masson. [ Google Scholar ]
- Centers for Disease Control and Prevention (2017). Reported Cases Of Notifiable Diseases And Rates Per 100,000, Excluding U.S. Territories, United States, 2017. Atlanta: Centers for Disease Control and Prevention. [ Google Scholar ]
- Chan J. F., Yuan S., Kok K. H., To K. K., Chu H., Yang J., et al. (2020). A familial cluster of pneumonia associated with the 2019 novel coronavirus indicating person-to-person transmission: a study of a family cluster. Lancet 395 514–523. [ PMC free article ] [ PubMed ] [ Google Scholar ]
- Chen M., Rodrigues C. M. C., Harrison O. B., Zhang C., Tan T., Chen J., et al. (2018). Invasive meningococcal disease in Shanghai, China from 1950 to 2016: implications for serogroup B vaccine implementation. Sci. Rep. 8 : 12334 . [ PMC free article ] [ PubMed ] [ Google Scholar ]
- Christenson B., Hedlund J., Lundbergh P., Ortqvist A. (2004). Additive preventive effect of influenza and pneumococcal vaccines in elderly persons. Eur. Resp. J. 23 363–368. [ PubMed ] [ Google Scholar ]
- Clark H. F., Offit P. A., Plotkin S. A., Heaton P. M. (2006). The new pentavalent rotavirus vaccine composed of bovine (strain WC3) -human rotavirus reassortants. Pediatr. Infect. Dis. J. 25 577–583. [ PubMed ] [ Google Scholar ]
- Curns A. T., Steiner C. A., Barrett M., Hunter K., Wilson E., Parashar U. D. (2010). Reduction in acute gastroenteritis hospitalizations among US children after introduction of rotavirus vaccine: analysis of hospital discharge data from 18 US states. J. Infect. Dis. 201 1617–1624. [ PubMed ] [ Google Scholar ]
- Daugla D. M., Gami J. P., Gamougam K., Naibei N., Mbainadji L., Narbe M., et al. (2014). Effect of a serogroup A meningococcal conjugate vaccine (PsA-TT) on serogroup A meningococcal meningitis and carriage in Chad: a community study [corrected]. Lancet 383 40–47. [ PMC free article ] [ PubMed ] [ Google Scholar ]
- Deogaonkar R., Hutubessy R., van der Putten I., Evers S., Jit M. (2012). Systematic review of studies evaluating the broader economic impact of vaccination in low and middle income countries. BMC Public Health 12 : 878 10.1186/s12916-017-0911-878 [ PMC free article ] [ PubMed ] [ CrossRef ] [ Google Scholar ]
- Dominguez A., Castilla J., Godoy P., Delgado-Rodriguez M., Saez M., Soldevila N., et al. (2013). Benefit of conjugate pneumococcal vaccination in preventing influenza hospitalization in children: a case-control study. Pediatr. Infect. Dis. J. 32 330–334. [ PubMed ] [ Google Scholar ]
- Donaghy M., Cameron J. C., Friederichs V. (2006). Increasing incidence of mumps in Scotland: options for reducing transmission. J. Clin. Virol. 35 121–129. [ PubMed ] [ Google Scholar ]
- Ehreth J. (2003). The global value of vaccination. Vaccine 21 596–600. [ PubMed ] [ Google Scholar ]
- Ferlay J., Soerjomataram I., Dikshit R., Eser S., Mathers C., Rebelo M., et al. (2012). Cancer incidence and mortality worldwide: sources, methods and major patterns in GLOBOCAN. Int. J. Cancer 136 E359–E386. [ PubMed ] [ Google Scholar ]
- Fine P. E., Griffiths U. K. (2007). Global poliomyelitis eradication: status and implications. Lancet 369 1321–1322. [ PubMed ] [ Google Scholar ]
- Fulop T., Larbi A., Dupuis G., Le Page A., Frost E. H., Cohen A. A., et al. (2017). Immunosenescence and inflamm-aging as two sides of the same coin: friends or foes? Front. Immunol. 8 : 1960 . 10.3389/fmicb.2018.01960 [ PMC free article ] [ PubMed ] [ CrossRef ] [ Google Scholar ]
- Funk S., Knapp J. K., Lebo E., Reef S. E., Dabbagh A. J., Kretsinger K., et al. (2019). Combining serological and contact data to derive target immunity levels for achieving and maintaining measles elimination. BMC Med. 17 : 180 10.1186/s12889-019-6655-180 [ PMC free article ] [ PubMed ] [ CrossRef ] [ Google Scholar ]
- Geddes A. M. (2006). The history of smallpox. Clin. Dermatol. 24 152–157. [ PubMed ] [ Google Scholar ]
- Gentile I., Borgia G. (2014). Vertical transmission of hepatitis B virus: challenges and solutions. Intern. J. Women Health 6 605–611. [ PMC free article ] [ PubMed ] [ Google Scholar ]
- Gessner B. D., Kaslow D., Louis J., Neuzil K., O’Brien K. L., Picot V., et al. (2017). Estimating the full public health value of vaccination. Vaccine 35 6255–6263. [ PubMed ] [ Google Scholar ]
- GHRF Commission (2016). Commission on a Global Health Risk Framework for the Future). The Neglected Dimension of Global Security A Framework to Counter Infectious Disease Crises. Commission on Global Health Risk Framework for the Future. Available online at: https://nam.edu/wp-content/uploads/2016/01/Neglected-Dimension-of-Global-Security.pdf (September 24, 2019). [ Google Scholar ]
- Glenny A. T., Hopkins B. E. (1923). Diphtheria toxoid as an immunising agent. Br. J. Exp. Pathol. 4 283–288. [ Google Scholar ]
- Global Polio Eradication Initiative (2019). Polio this Week, Wild Poliovirus Type 1 And Circulating Vaccine-Derived Poliovirus Cases. Available online at: http://polioeradication.org/polio-today/polio-now/this-week/ (accessed June 25, 2020). [ Google Scholar ]
- Gold R., Artenstein M. S. (1971). Meningococcal infections. 2. Field trial of group C meningococcal polysaccharide vaccine in 1969-70. Bull. World Health Organ. 45 279–282. [ PMC free article ] [ PubMed ] [ Google Scholar ]
- Gouglas D., Thanh, Le T., Henderson K., Kaloudis A., Danielsen T., et al. (2018). Estimating the cost of vaccine development against epidemic infectious diseases: a cost minimisation study. Lancet Glob. Health 6 : e001386-96 . [ PMC free article ] [ PubMed ] [ Google Scholar ]
- Grizas A. P., Camenga D., Vazquez M. (2012). Cocooning: a concept to protect young children from infectious diseases. Curr. Opin. Pediatr. 24 92–97. [ PubMed ] [ Google Scholar ]
- Gupta G., Glueck R., Patel P. R. (2017). HPV vaccines: global perspectives. Hum. Vacc. Immunotherap. 13 1–4. [ PMC free article ] [ PubMed ] [ Google Scholar ]
- Gupta M., Angeli F., Bosma H., Rana M., Prinja S., Kumar R., et al. (2016). Effectiveness of multiple-strategy community intervention in reducing geographical, socioeconomic and gender based inequalities in maternal and child health outcomes in Haryana, India. PLoS One 11 : e0150537 . 10.1371/journal.pone.0150537 [ PMC free article ] [ PubMed ] [ CrossRef ] [ Google Scholar ]
- Hajjeh R. (2011). Accelerating introduction of new vaccines: barriers to introduction and lessons learned from the recent Haemophilus influenzae type B vaccine experience. Philos. Trans. R. Soc. B. 366 2827–2832. [ PMC free article ] [ PubMed ] [ Google Scholar ]
- Hermans M. P. J., Kooistra J., Cannegieter S. C., Rosendaal F. R., Mook-Kanamori D. O., Nemeth B. (2017). Healthcare and disease burden among refugees in long-stay refugee camps at Lesbos. Greece. Eur. J. Epidemiol. 32 851–854. [ PubMed ] [ Google Scholar ]
- Heukelbach J., Alencar C. H., Kelvin A. A., de Oliveira W. K., Pamplona de Goes C. L. (2016). Zika virus outbreak in Brazil. J. Infect. Dev. Countr. 10 116–120. [ PubMed ] [ Google Scholar ]
- Hill H. A., Elam-Evans L. D., Yankey D., Singleton J. A., Kang Y. (2017). Vaccination coverage among children aged 19-35 Months - United States, 2016. MMWR Morb. Mort. Wkly Rep. 66 1171–1177. [ PMC free article ] [ PubMed ] [ Google Scholar ]
- Hinman A. R., McKinlay M. A. (2015). Immunization equity. Vaccine 33 ( Suppl. 4 ), D72–D77. [ PubMed ] [ Google Scholar ]
- Hotez P. J. (2001). Vaccines as instruments of foreign policy. The new vaccines for tropical infectious diseases may have unanticipated uses beyond fighting diseases. EMBO Rep. 2 862–868. [ PMC free article ] [ PubMed ] [ Google Scholar ]
- Janssens W. (2011). Externalities in program evaluation: the impact of a Women’s empowerment program on immunization. J. Eur. Econ. Assoc. 9 1082–1113. [ Google Scholar ]
- Jenner E. (1798). An Inquiry Into The Causes And Effects Of The Variolæ Vaccinæ, A Disease Discovered In Some Of The Western Counties Of England, Particularly Gloucestershire, And Known By The Name Of The Cow Pox. London: Sampson Low. [ Google Scholar ]
- Jit M., Hutubessy R., Png M. E., Sundaram N., Audimulam J., Salim S., et al. (2015). The broader economic impact of vaccination: reviewing and appraising the strength of evidence. BMC Med. 13 : 209 10.1186/s12916-017-0911-209 [ PMC free article ] [ PubMed ] [ CrossRef ] [ Google Scholar ]
- Keja K., Chan C., Hayden G., Henderson R. H. (1988). Expanded programme on immunization. World Health Stat. Q. 41 59–63. [ PubMed ] [ Google Scholar ]
- Kirnbauer R., Booy F., Cheng N., Lowy D. R., Schiller J. T. (1992). Papillomavirus L1 major capsid protein self-assembles into virus-like particles that are highly immunogenic. Proc. Nat. Acad. Sci. U.S.A. 89 12180–12184. [ PMC free article ] [ PubMed ] [ Google Scholar ]
- Kyaw M. H., Lynfield R., Schaffner W., Craig A. S., Hadler J., Reingold A., et al. (2006). Effect of introduction of the pneumococcal conjugate vaccine on drug-resistant Streptococcus pneumoniae . New Engl. J. Med. 354 1455–1463. [ PubMed ] [ Google Scholar ]
- Levine M. M., Robins-Browne R. (2009). Vaccines, global health and social equity. Immunol. Cell Biol. 87 274–278. [ PubMed ] [ Google Scholar ]
- Lien G., Heymann D. L. (2013). The problems with polio: toward eradication. Infect. Dis. Ther. 2 167–174. [ PMC free article ] [ PubMed ] [ Google Scholar ]
- Lieu T. A., Cochi S. L., Black S. B., Halloran M. E., Shinefield H. R., Holmes S. J., et al. (1994). Cost-effectiveness of a routine varicella vaccination program for US children. JAMA 271 375–381. [ PubMed ] [ Google Scholar ]
- Liu Y. Y., Wang Y., Walsh T. R., Yi L. X., Zhang R., Spencer J., et al. (2016). Emergence of plasmid-mediated colistin resistance mechanism MCR-1 in animals and human beings in China: a microbiological and molecular biological study. Lancet Infect. Dis. 16 161–168. [ PubMed ] [ Google Scholar ]
- Logan L. K., Weinstein R. A. (2017). The epidemiology of carbapenem-resistant Enterobacteriaceae : the impact and evolution of a global menace. J. Infect. Dis. 215 ( Suppl._1 ), S28–S36. [ PMC free article ] [ PubMed ] [ Google Scholar ]
- Maassab H. F., DeBorde D. C. (1985). Development and characterization of cold-adapted viruses for use as live virus vaccines. Vaccine 3 355–369. [ PubMed ] [ Google Scholar ]
- Madhi S. A., Klugman K. P., Vaccine Trialist G. (2004). A role for Streptococcus pneumoniae in virus-associated pneumonia. Nat. Med. 10 811–813. [ PMC free article ] [ PubMed ] [ Google Scholar ]
- Maiden M. C., Ibarz-Pavon A. B., Urwin R., Gray S. J., Andrews N. J., Clarke S. C., et al. (2008). Impact of meningococcal serogroup C conjugate vaccines on carriage and herd immunity. J. Infect. Dis. 197 737–743. [ PMC free article ] [ PubMed ] [ Google Scholar ]
- Manzoli L., Schioppa F., Boccia A., Villari P. (2007). The efficacy of influenza vaccine for healthy children: a meta-analysis evaluating potential sources of variation in efficacy estimates including study quality. Pediatr. Infect. Dis. J. 26 97–106. [ PubMed ] [ Google Scholar ]
- Marchant A., Sadarangani M., Garand M., Dauby N., Verhasselt V., Pereira L., et al. (2017). Maternal immunisation: collaborating with mother nature. Lancet. Infect. Dis. 17 e197–e208. 10.1016/S1473-3099(17)30229-3 [ PubMed ] [ CrossRef ] [ Google Scholar ]
- Mina M. J., Kula T., Leng Y., Li M., de Vries R. D., Knip M., et al. (2019). Measles virus infection diminishes preexisting antibodies that offer protection from other pathogens. Science 366 599–606. [ PMC free article ] [ PubMed ] [ Google Scholar ]
- Mina M. J., Metcalf C. J., de Swart R. L., Osterhaus A. D., Grenfell B. T. (2015). Long-term measles-induced immunomodulation increases overall childhood infectious disease mortality. Science 348 694–699. [ PMC free article ] [ PubMed ] [ Google Scholar ]
- Morens D. M., Fauci A. S. (2007). The 1918 influenza pandemic: insights for the 21st century. J. Infect. Dis. 195 1018–1028. [ PubMed ] [ Google Scholar ]
- Morens D. M., Holmes E. C., Davis A. S., Taubenberger J. K. (2011). Global rinderpest eradication: lessons learned and why humans should celebrate too. J. Infect. Dis. 204 502–505. [ PMC free article ] [ PubMed ] [ Google Scholar ]
- Moss W. J. (2017). Measles. Lancet 390 2490–2502. [ PubMed ] [ Google Scholar ]
- Munira S. L., Fritzen S. A. (2007). What influences government adoption of vaccines in developing countries? A policy process analysis. Soc. Sci. Med. 65 1751–1764. [ PubMed ] [ Google Scholar ]
- Needham J. (2000). Science and Civilisation in China: Volume 6, Biology and Biological Technology. Cambridge: Cambridge University Press. [ Google Scholar ]
- Niessen L. W., ten Hove A., Hilderink H., Weber M., Mulholland K., Ezzati M. (2009). Comparative impact assessment of child pneumonia interventions. Bull. World Health Organ. 87 472–480. [ PMC free article ] [ PubMed ] [ Google Scholar ]
- Nunes M. C., Cutland C. L., Jones S., Downs S., Weinberg A., Ortiz J. R., et al. (2017). Efficacy of maternal influenza vaccination against all-cause lower respiratory tract infection hospitalizations in young infants: results from a randomized controlled trial. Clin. Infect. Dis. 65 1066–1071. [ PMC free article ] [ PubMed ] [ Google Scholar ]
- Orenstein W. A., Ahmed R. (2017). Simply put: vaccination saves lives. Proc. Nat. Acad. Sci. U.S.A. 114 4031–4033. [ PMC free article ] [ PubMed ] [ Google Scholar ]
- Ozawa S., Clark S., Portnoy A., Grewal S., Brenzel L., Walker D. G. (2016). Return On investment from childhood immunization in low- and middle-income countries, 2011-20. Health Aff. 35 199–207. [ PubMed ] [ Google Scholar ]
- Palmer T., Wallace L., Pollock K. G., Cuschieri K., Robertson C., Kavanagh K., et al. (2019). Prevalence of cervical disease at age 20 after immunisation with bivalent HPV vaccine at age 12-13 in Scotland: retrospective population study. BMJ 365 : l1161 . [ PMC free article ] [ PubMed ] [ Google Scholar ]
- Palmu A. A., Jokinen J., Nieminen H., Rinta-Kokko H., Ruokokoski E., Puumalainen T., et al. (2014). Effect of pneumococcal Haemophilus influenzae protein D conjugate vaccine (PHiD-CV10) on outpatient antimicrobial purchases: a double-blind, cluster randomised phase 3-4 trial. Lancet Infect. Dis. 14 205–212. [ PubMed ] [ Google Scholar ]
- Pasteur L. (1880). De l’attenuation du virus du choléra des poules. Comptes Rendus De l’Acad. Sci. 91 673–680. [ Google Scholar ]
- Pasteur L. (1881). Sur la vaccination charbonneuse. Comptes Rendus De l’Acad. Sci. 92 1378–1383. [ Google Scholar ]
- Pasteur L. (1885). Mèthode pour prévenir la rage apres morsure. Comptes Rendus De l’Acad. Sci. 101 765–772. [ Google Scholar ]
- Paulke-Korinek M., Kundi M., Rendi-Wagner P., de Martin A., Eder G., Schmidle-Loss B., et al. (2011). Herd immunity after two years of the universal mass vaccination program against rotavirus gastroenteritis in Austria. Vaccine 29 2791–2796. [ PubMed ] [ Google Scholar ]
- Peltola H., Aavitsland P., Hansen K. G., Jonsdottir K. E., Nokleby H., Romanus V. (1999). Perspective: a five-country analysis of the impact of four different Haemophilus influenzae type b conjugates and vaccination strategies in Scandinavia. J. Infect. Dis. 179 223–229. [ PubMed ] [ Google Scholar ]
- Petrova V. N., Sawatsky B., Han A. X., Laksono B. M., Walz L., Parker E., et al. (2019). Incomplete genetic reconstitution of B cell pools contributes to prolonged immunosuppression after measles. Sci. Immunol. 4 : eaay6125 . [ PubMed ] [ Google Scholar ]
- Plans-Rubio P. (2012). Evaluation of the establishment of herd immunity in the population by means of serological surveys and vaccination coverage. Hum. Vacc. Immunotherap. 8 184–188. [ PubMed ] [ Google Scholar ]
- Plotkin S. (2014). History of vaccination. Proc. Natl. Acad. Sci. U.S.A. 111 12283–12287. [ PMC free article ] [ PubMed ] [ Google Scholar ]
- Plotkin S. A. (2017). Vaccines for epidemic infections and the role of CEPI. Hum. Vacc. Immunotherap. 13 2755–2762. [ PMC free article ] [ PubMed ] [ Google Scholar ]
- Plotkin S. A., Mortimer E. A. (1988). Vaccines. Philadelphia, PA: Saunders. [ Google Scholar ]
- Pollard A. J., Perrett K. P., Beverley P. C. (2009). Maintaining protection against invasive bacteria with protein-polysaccharide conjugate vaccines. Nat. Rev. Immunol. 9 213–220. [ PubMed ] [ Google Scholar ]
- Poovorawan Y., Chongsrisawat V., Theamboonlers A., Leroux-Roels G., Kuriyakose S., Leyssen M., et al. (2011). Evidence of protection against clinical and chronic hepatitis B infection 20 years after infant vaccination in a high endemicity region. J. Viral Hepat. 18 369–375. [ PMC free article ] [ PubMed ] [ Google Scholar ]
- Public Health England (2018). Public Health Matters Ten Years On Since The Start Of The HPV Vaccine Programme – What Impact Is It Having? London: Public Health England. [ Google Scholar ]
- Public Health England (2019). Human Papillomavirus (HPV) Vaccination Coverage In Adolescent Females In England: 2017/18. London: Public Health England. [ Google Scholar ]
- Regan A. K., de Klerk N., Moore H. C., Omer S. B., Shellam G., Effler P. V. (2016). Effect of maternal influenza vaccination on hospitalization for respiratory infections in newborns: a retrospective cohort study. Pediatr. Infect. Dis. J. 35 1097–1103. [ PubMed ] [ Google Scholar ]
- Riumallo-Herl C., Chang A. Y., Clark S., Constenla D., Clark A., Brenzel L., et al. (2018). Poverty reduction and equity benefits of introducing or scaling up measles, rotavirus and pneumococcal vaccines in low-income and middle-income countries: a modelling study. BMJ Glob. Health 3 : e000613 . [ PMC free article ] [ PubMed ] [ Google Scholar ]
- Roeder P., Mariner J., Kock R. (2013). Rinderpest: the veterinary perspective on eradication. Philos. Trans. R. Soc. B 368 : 20120139 . [ PMC free article ] [ PubMed ] [ Google Scholar ]
- Roush S. W., Murphy T. V. (2007). Vaccine-preventable disease table working G. historical comparisons of morbidity and mortality for vaccine-preventable diseases in the United States. JAMA 298 2155–2163. [ PubMed ] [ Google Scholar ]
- Russo F. B., Jungmann P., Beltrao-Braga P. C. B. (2017). Zika infection and the development of neurological defects. Cell. Microbiol. 19 : 12744. [ PubMed ] [ Google Scholar ]
- Sah R. K. (1991). The effects of child-mortality changes on fertility choice and parental welfare. J. Polit. Econ. 99 582–606. [ Google Scholar ]
- Sato Y., Sato H. (1999). Development of acellular pertussis vaccines. Biologicals 27 61–69. [ PubMed ] [ Google Scholar ]
- Schneerson R., Barrera O., Sutton A., Robbins J. B. (1980). Preparation, characterization, and immunogenicity of Haemophilus influenzae type b polysaccharide-protein conjugates. J. Exp. Med. 152 361–376. [ PMC free article ] [ PubMed ] [ Google Scholar ]
- Serruto D., Bottomley M. J., Ram S., Giuliani M. M., Rappuoli R. (2012). The new multicomponent vaccine against meningococcal serogroup B, 4CMenB: immunological, functional and structural characterization of the antigens. Vaccine 30 ( Suppl. 2 ), B87–B97. [ PMC free article ] [ PubMed ] [ Google Scholar ]
- Shearley A. E. (1999). The societal value of vaccination in developing countries. Vaccine 17 ( Suppl. 3 ), S109–S112. [ PubMed ] [ Google Scholar ]
- Simonsen L., Taylor R. J., Young-Xu Y., Haber M., May L., Klugman K. P. (2011). Impact of pneumococcal conjugate vaccination of infants on pneumonia and influenza hospitalization and mortality in all age groups in the United States. mBio 2 : e00309-10 . [ PMC free article ] [ PubMed ] [ Google Scholar ]
- Soergel P., Makowski L., Schippert C., Staboulidou I., Hille U., Hillemanns P. (2012). The cost efficiency of HPV vaccines is significantly underestimated due to omission of conisation-associated prematurity with neonatal mortality and morbidity. Hum. Vacc. Immunother. 8 243–251. [ PubMed ] [ Google Scholar ]
- Strassburg M. A. (1982). The global eradication of smallpox. Am. J. Infect. Control 10 53–59. [ PubMed ] [ Google Scholar ]
- Tabrizi S. N., Brotherton J. M., Kaldor J. M., Skinner S. R., Liu B., Bateson D., et al. (2014). Assessment of herd immunity and cross-protection after a human papillomavirus vaccination programme in Australia: a repeat cross-sectional study. Lancet Infect.ous Dis. 14 958–966. [ PubMed ] [ Google Scholar ]
- Thacker N., Vashishtha V. M., Thacker D. (2016). Polio eradication in India: the lessons learned. Pediatrics 138 : e20160461 . [ PubMed ] [ Google Scholar ]
- Thanh Le T., Andreadakis Z., Kumar A., Gomez Roman R., Tollefsen S., Saville M., et al. (2020). The COVID-19 vaccine development landscape. Nat. Rev. Drug Discov. 19 305–306. [ PubMed ] [ Google Scholar ]
- Theiler M., Smith H. H. (1937a). The effect of prolonged cultivation in vitro upon the pathogenicity of yellow fever virus. J. Exp. Med. 65 767–786. [ PMC free article ] [ PubMed ] [ Google Scholar ]
- Theiler M., Smith H. H. (1937b). The use of yellow fever virus modified by in vitro cultivation for human immunization. J. Exp. Med. 65 787–800. [ PMC free article ] [ PubMed ] [ Google Scholar ]
- Turner H. C., Thwaites G. E., Clapham H. E. (2018). Vaccine-preventable diseases in lower-middle-income countries. Lancet Infect. Dis. 18 937–939. [ PubMed ] [ Google Scholar ]
- United Nations Department of Economic and Social Affairs (2019). Population Division. World Population Prospects 2019. New York, NY: United Nations Department of Economic and Social Affairs. [ Google Scholar ]
- Van der Wielen M., Giaquinto C., Gothefors L., Huelsse C., Huet F., Littmann M., et al. (2010). Impact of community-acquired paediatric rotavirus gastroenteritis on family life: data from the REVEAL study. BMC Fam. Pract. 11 : 22 10.1186/s12916-017-0911-22 [ PMC free article ] [ PubMed ] [ CrossRef ] [ Google Scholar ]
- Verguet S., Murphy S., Anderson B., Johansson K. A., Glass R., Rheingans R. (2013). Public finance of rotavirus vaccination in India and Ethiopia: an extended cost-effectiveness analysis. Vaccine 31 4902–4910. [ PubMed ] [ Google Scholar ]
- Wang X., Huang X., Zhang Y. (2018). Involvement of human papillomaviruses in cervical cancer. Front. Microbiol. 9 : 2896 . 10.3389/fmicb.2018.02896 [ PMC free article ] [ PubMed ] [ CrossRef ] [ Google Scholar ]
- Whitney C. G., Farley M. M., Hadler J., Harrison L. H., Bennett N. M., Lynfield R., et al. (2003). Decline in invasive pneumococcal disease after the introduction of protein-polysaccharide conjugate vaccine. New Engl. J. Med. 348 1737–1746. [ PubMed ] [ Google Scholar ]
- Wilder-Smith A., Longini I., Zuber P. L., Barnighausen T., Edmunds W. J., Dean N., et al. (2017). The public health value of vaccines beyond efficacy: methods, measures and outcomes. BMC Med. 15 : 138 . 10.1186/s12916-017-0911-8 [ PMC free article ] [ PubMed ] [ CrossRef ] [ Google Scholar ]
- Wilson R., Paterson P., Larson H. J. (2019). Strategies to improve maternal vaccination acceptance. BMC Public Health 19 : 342 . 10.1186/s12889-019-6655-y [ PMC free article ] [ PubMed ] [ CrossRef ] [ Google Scholar ]
- Wise J. (2018). Child vaccination rates drop in England as MMR uptake falls for fourth year. BMJ 362 : k3967 . [ PubMed ] [ Google Scholar ]
- Wise J. (2019). MMR vaccine: johnson urges new impetus to increase uptake as UK loses measles-free status. BMJ 366 : l5219 . [ PubMed ] [ Google Scholar ]
- World Bank (2019). Immunization, DPT (% of Children Ages 12-23 Months). Washington, DC: World Bank. [ Google Scholar ]
- World Health Assembly (1974). The expanded programme on immunization: the 1974 resolution by the world health assembly. Assign. Child. 1985 87–88. [ PubMed ] [ Google Scholar ]
- World Health Organisation (2019a). Immunization Coverage. Geneva: World Health Organisation. [ Google Scholar ]
- World Health Organisation (2019b). Polio Eradication. Geneva: World Health Organisation. [ Google Scholar ]
- World Health Organisation (2019c). Reported Cases of Selected Vaccine Preventable Diseases (VPDs). Geneva: World Health Organisation. [ Google Scholar ]
- World Health Organization [WHO], United Nations Children’s Fund [UNICEF] (2019). WHO/UNICEF Estimates of National Immunization Coverage (WUENIC) 1980-2018. Geneva: WHO. [ Google Scholar ]
- Yezli S., Gautret P., Assiri A. M., Gessner B. D., Alotaibi B. (2018). Prevention of meningococcal disease at mass gatherings: lessons from the Hajj and Umrah. Vaccine 36 4603–4609. [ PubMed ] [ Google Scholar ]
- Zhou F., Shefer A., Wenger J., Messonnier M., Wang L. Y., Lopez A., et al. (2009). Economic evaluation of the routine childhood immunization program in the United States. Pediatrics 133 577–585. [ PubMed ] [ Google Scholar ]
- Zhu N., Zhang D., Wang W., Li X., Yang B., Song J., et al. (2020). A novel coronavirus from patients with pneumonia in China, 2019. New Engl. J. Med. 382 727–733. [ PMC free article ] [ PubMed ] [ Google Scholar ]
Thank you for visiting nature.com. You are using a browser version with limited support for CSS. To obtain the best experience, we recommend you use a more up to date browser (or turn off compatibility mode in Internet Explorer). In the meantime, to ensure continued support, we are displaying the site without styles and JavaScript.
- View all journals
Vaccines articles from across Nature Portfolio
Vaccines are a clinical product that is composed of live or dead material from an infectious agent – bacterium, virus, fungus or parasite – that elicit protective immunity against the pathogen when administered. These substances are used to prevent the spread of infectious diseases.
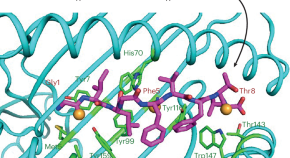
Peptide vaccines get an OS update
Peptide vaccines use antigenic peptide fragments to induce an immune response but are problematic because of the short half-life of peptides. A study now reports thioamide substitution in the peptide backbone as a strategy to enhance resistance to proteolysis and promote binding to the MHC I complex for T cell activation.
- Martin Zacharias
- Sebastian Springer
Related Subjects
- Cell vaccines
- Conjugate vaccines
- DNA vaccines
- Inactivated vaccines
- Live attenuated vaccines
- Peptide vaccines
- Protein vaccines
- RNA vaccines
Latest Research and Reviews
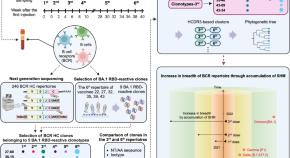
An ancestral SARS-CoV-2 vaccine induces anti-Omicron variants antibodies by hypermutation
Repeat vaccination with COVID-19 mRNA vaccines has been shown to increase breadth of the antibody response. Here the authors demonstrate that B cell clones induced by the ancestral COVID-19 vaccine develop into daughter clones with different reactivity to individual SARS-CoV-2 variants through the accumulation of somatic hypermutations.
- Seoryeong Park
- Jaewon Choi
- Junho Chung
Strep A: challenges, opportunities, vaccine-based solutions, and economics
- David E. Bloom
- Jonathan Carapetis
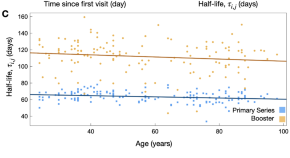
SARS-CoV-2 booster vaccine dose significantly extends humoral immune response half-life beyond the primary series
- Chapin S. Korosec
- David W. Dick
- James Watmough
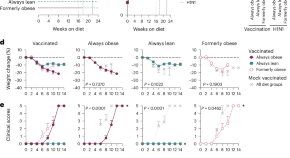
Diet switch pre-vaccination improves immune response and metabolic status in formerly obese mice
Diet switching from high-fat to standard diet before influenza vaccination affects the metabolic state of T cells, restores their responses and improves vaccine efficacy in mice.
- Rebekah Honce
- Ana Vazquez-Pagan
- Stacey Schultz-Cherry
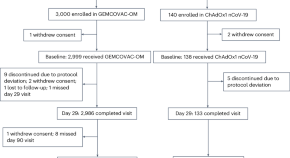
An Omicron-specific, self-amplifying mRNA booster vaccine for COVID-19: a phase 2/3 randomized trial
A self-amplifying mRNA vaccine shows promise in this new modality by eliciting neutralizing antibodies against the SARS-CoV-2 Omicron (BA.1) variant in a phase 2/3 trial.
- Rohan Gurjar
- Vijaykumar Barge
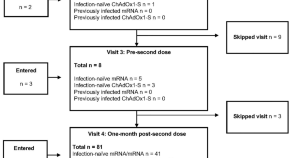
Adaptive immune responses to two-dose COVID-19 vaccine series in healthy Canadian adults ≥ 50 years: a prospective, observational cohort study
- Gabrielle N. Gaultier
- Brynn McMillan
- Manish Sadarangani
News and Comment
The role of correlates of protection in overcoming barriers to vaccine development and demonstrating efficacy.
- Deborah F. King
- Helen Groves
- Charlotte Weller
Facilitating broad antibody responses
- Laurie A. Dempsey
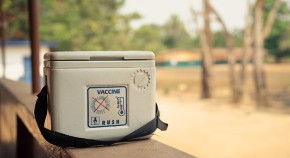
Going the extra mile to increase vaccine uptake
Deployment of mobile vaccination teams to remote communities in Sierra Leone substantially increased COVID-19 vaccine uptake, and could potentially be bundled with other health interventions.
- Karen O’Leary
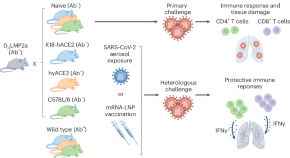
The power of memory T cells minus antibodies
T cell- and antibody-based immunological protection are generally considered to function together, but data now show how T cells conferred by previous SARS-CoV-2 infection or two-dose vaccination can elicit heterologous protection in mice against subsequent SARS-CoV-2 infection, even in the absence of antibodies.
- Thi H. O. Nguyen
- Katherine Kedzierska
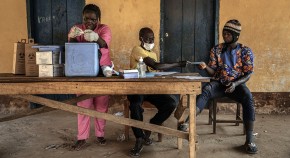
Mobile delivery of COVID-19 vaccines improved uptake in rural Sierra Leone
A trial that took mobile health services to rural Sierra Leone finds that this initiative increased COVID-19 vaccine uptake. But more must be done to expand the coverage of health services in low-income countries.
- Alison Buttenheim
- Harsha Thirumurthy
Quick links
- Explore articles by subject
- Guide to authors
- Editorial policies

Numbers, Facts and Trends Shaping Your World
Read our research on:
Full Topic List
Regions & Countries
- Publications
- Our Methods
- Short Reads
- Tools & Resources
Read Our Research On:
How Pew Research Center will report on generations moving forward
Journalists, researchers and the public often look at society through the lens of generation, using terms like Millennial or Gen Z to describe groups of similarly aged people. This approach can help readers see themselves in the data and assess where we are and where we’re headed as a country.
Pew Research Center has been at the forefront of generational research over the years, telling the story of Millennials as they came of age politically and as they moved more firmly into adult life . In recent years, we’ve also been eager to learn about Gen Z as the leading edge of this generation moves into adulthood.
But generational research has become a crowded arena. The field has been flooded with content that’s often sold as research but is more like clickbait or marketing mythology. There’s also been a growing chorus of criticism about generational research and generational labels in particular.
Recently, as we were preparing to embark on a major research project related to Gen Z, we decided to take a step back and consider how we can study generations in a way that aligns with our values of accuracy, rigor and providing a foundation of facts that enriches the public dialogue.
A typical generation spans 15 to 18 years. As many critics of generational research point out, there is great diversity of thought, experience and behavior within generations.
We set out on a yearlong process of assessing the landscape of generational research. We spoke with experts from outside Pew Research Center, including those who have been publicly critical of our generational analysis, to get their take on the pros and cons of this type of work. We invested in methodological testing to determine whether we could compare findings from our earlier telephone surveys to the online ones we’re conducting now. And we experimented with higher-level statistical analyses that would allow us to isolate the effect of generation.
What emerged from this process was a set of clear guidelines that will help frame our approach going forward. Many of these are principles we’ve always adhered to , but others will require us to change the way we’ve been doing things in recent years.
Here’s a short overview of how we’ll approach generational research in the future:
We’ll only do generational analysis when we have historical data that allows us to compare generations at similar stages of life. When comparing generations, it’s crucial to control for age. In other words, researchers need to look at each generation or age cohort at a similar point in the life cycle. (“Age cohort” is a fancy way of referring to a group of people who were born around the same time.)
When doing this kind of research, the question isn’t whether young adults today are different from middle-aged or older adults today. The question is whether young adults today are different from young adults at some specific point in the past.
To answer this question, it’s necessary to have data that’s been collected over a considerable amount of time – think decades. Standard surveys don’t allow for this type of analysis. We can look at differences across age groups, but we can’t compare age groups over time.
Another complication is that the surveys we conducted 20 or 30 years ago aren’t usually comparable enough to the surveys we’re doing today. Our earlier surveys were done over the phone, and we’ve since transitioned to our nationally representative online survey panel , the American Trends Panel . Our internal testing showed that on many topics, respondents answer questions differently depending on the way they’re being interviewed. So we can’t use most of our surveys from the late 1980s and early 2000s to compare Gen Z with Millennials and Gen Xers at a similar stage of life.
This means that most generational analysis we do will use datasets that have employed similar methodologies over a long period of time, such as surveys from the U.S. Census Bureau. A good example is our 2020 report on Millennial families , which used census data going back to the late 1960s. The report showed that Millennials are marrying and forming families at a much different pace than the generations that came before them.
Even when we have historical data, we will attempt to control for other factors beyond age in making generational comparisons. If we accept that there are real differences across generations, we’re basically saying that people who were born around the same time share certain attitudes or beliefs – and that their views have been influenced by external forces that uniquely shaped them during their formative years. Those forces may have been social changes, economic circumstances, technological advances or political movements.
When we see that younger adults have different views than their older counterparts, it may be driven by their demographic traits rather than the fact that they belong to a particular generation.
The tricky part is isolating those forces from events or circumstances that have affected all age groups, not just one generation. These are often called “period effects.” An example of a period effect is the Watergate scandal, which drove down trust in government among all age groups. Differences in trust across age groups in the wake of Watergate shouldn’t be attributed to the outsize impact that event had on one age group or another, because the change occurred across the board.
Changing demographics also may play a role in patterns that might at first seem like generational differences. We know that the United States has become more racially and ethnically diverse in recent decades, and that race and ethnicity are linked with certain key social and political views. When we see that younger adults have different views than their older counterparts, it may be driven by their demographic traits rather than the fact that they belong to a particular generation.
Controlling for these factors can involve complicated statistical analysis that helps determine whether the differences we see across age groups are indeed due to generation or not. This additional step adds rigor to the process. Unfortunately, it’s often absent from current discussions about Gen Z, Millennials and other generations.
When we can’t do generational analysis, we still see value in looking at differences by age and will do so where it makes sense. Age is one of the most common predictors of differences in attitudes and behaviors. And even if age gaps aren’t rooted in generational differences, they can still be illuminating. They help us understand how people across the age spectrum are responding to key trends, technological breakthroughs and historical events.
Each stage of life comes with a unique set of experiences. Young adults are often at the leading edge of changing attitudes on emerging social trends. Take views on same-sex marriage , for example, or attitudes about gender identity .
Many middle-aged adults, in turn, face the challenge of raising children while also providing care and support to their aging parents. And older adults have their own obstacles and opportunities. All of these stories – rooted in the life cycle, not in generations – are important and compelling, and we can tell them by analyzing our surveys at any given point in time.
When we do have the data to study groups of similarly aged people over time, we won’t always default to using the standard generational definitions and labels. While generational labels are simple and catchy, there are other ways to analyze age cohorts. For example, some observers have suggested grouping people by the decade in which they were born. This would create narrower cohorts in which the members may share more in common. People could also be grouped relative to their age during key historical events (such as the Great Recession or the COVID-19 pandemic) or technological innovations (like the invention of the iPhone).
By choosing not to use the standard generational labels when they’re not appropriate, we can avoid reinforcing harmful stereotypes or oversimplifying people’s complex lived experiences.
Existing generational definitions also may be too broad and arbitrary to capture differences that exist among narrower cohorts. A typical generation spans 15 to 18 years. As many critics of generational research point out, there is great diversity of thought, experience and behavior within generations. The key is to pick a lens that’s most appropriate for the research question that’s being studied. If we’re looking at political views and how they’ve shifted over time, for example, we might group people together according to the first presidential election in which they were eligible to vote.
With these considerations in mind, our audiences should not expect to see a lot of new research coming out of Pew Research Center that uses the generational lens. We’ll only talk about generations when it adds value, advances important national debates and highlights meaningful societal trends.
- Age & Generations
- Demographic Research
- Generation X
- Generation Z
- Generations
- Greatest Generation
- Methodological Research
- Millennials
- Silent Generation
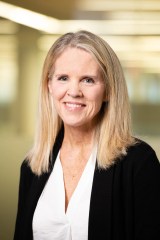
How Teens and Parents Approach Screen Time
Who are you the art and science of measuring identity, u.s. centenarian population is projected to quadruple over the next 30 years, older workers are growing in number and earning higher wages, teens, social media and technology 2023, most popular.
1615 L St. NW, Suite 800 Washington, DC 20036 USA (+1) 202-419-4300 | Main (+1) 202-857-8562 | Fax (+1) 202-419-4372 | Media Inquiries
Research Topics
- Coronavirus (COVID-19)
- Economy & Work
- Family & Relationships
- Gender & LGBTQ
- Immigration & Migration
- International Affairs
- Internet & Technology
- News Habits & Media
- Non-U.S. Governments
- Other Topics
- Politics & Policy
- Race & Ethnicity
- Email Newsletters
ABOUT PEW RESEARCH CENTER Pew Research Center is a nonpartisan fact tank that informs the public about the issues, attitudes and trends shaping the world. It conducts public opinion polling, demographic research, media content analysis and other empirical social science research. Pew Research Center does not take policy positions. It is a subsidiary of The Pew Charitable Trusts .
Copyright 2024 Pew Research Center
Terms & Conditions
Privacy Policy
Cookie Settings
Reprints, Permissions & Use Policy
- Download PDF
- Share X Facebook Email LinkedIn
- Permissions
Fatal Traffic Risks With a Total Solar Eclipse in the US
- 1 Department of Medicine, University of Toronto, Toronto, Ontario, Canada
- 2 Evaluative Clinical Science Platform, Sunnybrook Research Institute, Toronto, Ontario, Canada
- 3 Institute for Clinical Evaluative Sciences, Toronto, Ontario, Canada
- 4 Division of General Internal Medicine, Sunnybrook Health Sciences Centre, Toronto, Ontario, Canada
- 5 Center for Leading Injury Prevention Practice Education & Research, Toronto, Ontario, Canada
- 6 Department of Medicine, University of British Columbia, Vancouver, British Columbia, Canada
- 7 Centre for Clinical Epidemiology & Evaluation, University of British Columbia, Vancouver, British Columbia, Canada
A total solar eclipse occurs when the moon temporarily obscures the sun and casts a dark shadow across the earth. This astronomical spectacle has been described for more than 3 millennia and can be predicted with high precision. Eclipse-related solar retinopathy (vision loss from staring at the sun) is an established medical complication; however, other medical outcomes have received little attention. 1
Read More About
Redelmeier DA , Staples JA. Fatal Traffic Risks With a Total Solar Eclipse in the US. JAMA Intern Med. Published online March 25, 2024. doi:10.1001/jamainternmed.2023.5234
Manage citations:
© 2024
Artificial Intelligence Resource Center
Best of JAMA Network 2022
Browse and subscribe to JAMA Network podcasts!
Others Also Liked
Select your interests.
Customize your JAMA Network experience by selecting one or more topics from the list below.
- Academic Medicine
- Acid Base, Electrolytes, Fluids
- Allergy and Clinical Immunology
- American Indian or Alaska Natives
- Anesthesiology
- Anticoagulation
- Art and Images in Psychiatry
- Artificial Intelligence
- Assisted Reproduction
- Bleeding and Transfusion
- Caring for the Critically Ill Patient
- Challenges in Clinical Electrocardiography
- Climate and Health
- Climate Change
- Clinical Challenge
- Clinical Decision Support
- Clinical Implications of Basic Neuroscience
- Clinical Pharmacy and Pharmacology
- Complementary and Alternative Medicine
- Consensus Statements
- Coronavirus (COVID-19)
- Critical Care Medicine
- Cultural Competency
- Dental Medicine
- Dermatology
- Diabetes and Endocrinology
- Diagnostic Test Interpretation
- Drug Development
- Electronic Health Records
- Emergency Medicine
- End of Life, Hospice, Palliative Care
- Environmental Health
- Equity, Diversity, and Inclusion
- Facial Plastic Surgery
- Gastroenterology and Hepatology
- Genetics and Genomics
- Genomics and Precision Health
- Global Health
- Guide to Statistics and Methods
- Hair Disorders
- Health Care Delivery Models
- Health Care Economics, Insurance, Payment
- Health Care Quality
- Health Care Reform
- Health Care Safety
- Health Care Workforce
- Health Disparities
- Health Inequities
- Health Policy
- Health Systems Science
- History of Medicine
- Hypertension
- Images in Neurology
- Implementation Science
- Infectious Diseases
- Innovations in Health Care Delivery
- JAMA Infographic
- Law and Medicine
- Leading Change
- Less is More
- LGBTQIA Medicine
- Lifestyle Behaviors
- Medical Coding
- Medical Devices and Equipment
- Medical Education
- Medical Education and Training
- Medical Journals and Publishing
- Mobile Health and Telemedicine
- Narrative Medicine
- Neuroscience and Psychiatry
- Notable Notes
- Nutrition, Obesity, Exercise
- Obstetrics and Gynecology
- Occupational Health
- Ophthalmology
- Orthopedics
- Otolaryngology
- Pain Medicine
- Palliative Care
- Pathology and Laboratory Medicine
- Patient Care
- Patient Information
- Performance Improvement
- Performance Measures
- Perioperative Care and Consultation
- Pharmacoeconomics
- Pharmacoepidemiology
- Pharmacogenetics
- Pharmacy and Clinical Pharmacology
- Physical Medicine and Rehabilitation
- Physical Therapy
- Physician Leadership
- Population Health
- Primary Care
- Professional Well-being
- Professionalism
- Psychiatry and Behavioral Health
- Public Health
- Pulmonary Medicine
- Regulatory Agencies
- Reproductive Health
- Research, Methods, Statistics
- Resuscitation
- Rheumatology
- Risk Management
- Scientific Discovery and the Future of Medicine
- Shared Decision Making and Communication
- Sleep Medicine
- Sports Medicine
- Stem Cell Transplantation
- Substance Use and Addiction Medicine
- Surgical Innovation
- Surgical Pearls
- Teachable Moment
- Technology and Finance
- The Art of JAMA
- The Arts and Medicine
- The Rational Clinical Examination
- Tobacco and e-Cigarettes
- Translational Medicine
- Trauma and Injury
- Treatment Adherence
- Ultrasonography
- Users' Guide to the Medical Literature
- Vaccination
- Venous Thromboembolism
- Veterans Health
- Women's Health
- Workflow and Process
- Wound Care, Infection, Healing
- Register for email alerts with links to free full-text articles
- Access PDFs of free articles
- Manage your interests
- Save searches and receive search alerts
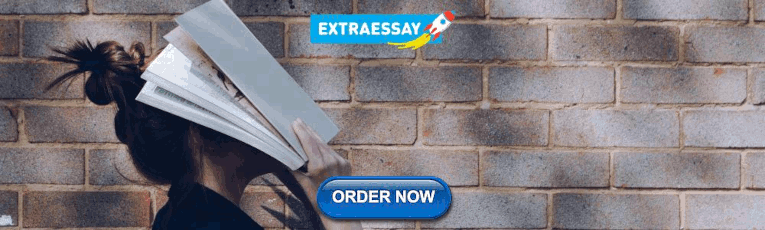
IMAGES
VIDEO
COMMENTS
A two-dose regimen of BNT162b2 (30 μg per dose, given 21 days apart) was found to be safe and 95% effective against Covid-19. The vaccine met both primary efficacy end points, with more than a 99 ...
Since the outbreak of the COVID-19 pandemic, there has been a rapid expansion in vaccine research focusing on exploiting the novel discoveries on the pathophysiology, genomics, and molecular biology of the severe acute respiratory syndrome coronavirus 2 (SARS-CoV-2) infection. Although the current preventive measures are primarily socially ...
Despite questions remain about the impact of virus variants and the duration of the immune response, messenger RNA (mRNA)-based and adenoviral vectored vaccines have demonstrated an overall efficacy from 70 to 95% in both phase III trials and real life. In addition, all these vaccines also reduce the severe forms of the disease and might ...
2. Vaccination strategies. Many efforts have been directed towards the development of the vaccines against COVID-19, to avert the pandemic and most of the developing vaccine candidates have been using the S-protein of SARS-CoV-2 (Dhama et al., 2020).As of July 2, 2020, the worldwide SARS-CoV-2 vaccine landscape includes 158 vaccine candidates, out of which 135 are in the preclinical or the ...
There is no question that the current vaccines are effective and safe. The risk of severe reaction to a COVID-19 jab, say researchers, is outweighed by the protection it offers against the deadly ...
The effectiveness of the mRNA vaccines in preventing COVID-19 disease progression in 2021 set new expectations about the role of prevention interventions for the disease. Efficacy observed in the trials was more than 90%.1,2 The efficacy of other vaccines evaluated in large randomised trials, such as the Oxford-AstraZeneca (70%) and Sputnik V (91%) vaccines, have been criticised for elements ...
Metrics. Vaccines have revolutionized modern medicine by preventing infectious diseases and safeguarding public health. This Collection showcases cutting-edge research on advancements in vaccine ...
Tetanus vaccines: WHO position paper, February 2017 — recommendations. Vaccine 36, 3573-3575 ... (SAGE) and a National Institute for Health Research (NIHR) Senior Investigator. The views ...
WASHINGTON — A new report from the National Academies of Sciences, Engineering, and Medicine reviews evidence for 19 potential harms of the COVID-19 vaccines, and for nine potential shoulder injuries from intramuscular administration of vaccines more broadly. The committee that conducted the review identified sufficient evidence to draw 20 conclusions about whether these vaccines could cause ...
Our analyses indicate that vaccine effectiveness generally decreases over time against SARS-CoV-2 infections, hospitalisations, and mortality. The baseline vaccine effectiveness levels for the omicron variant were notably lower than for other variants. Therefore, other preventive measures (eg, face-mask wearing and physical distancing) might be necessary to manage the pandemic in the long term.
To date, coronavirus disease 2019 (COVID-19) becomes increasingly fierce due to the emergence of variants. Rapid herd immunity through vaccination is needed to block the mutation and prevent the emergence of variants that can completely escape the immune surveillance. We aimed to systematically evaluate the effectiveness and safety of COVID-19 vaccines in the real world and to establish a ...
Vaccines are a public health success story, as they have prevented or lessened the effects of many infectious diseases. To address concerns around potential vaccine injuries, the Health Resources and Services Administration (HRSA) administers the Vaccine Injury Compensation Program (VICP) and the Countermeasures Injury Compensation Program (CICP), which provide compensation to those who assert ...
6 Clinical Excellence Research Center, School of Medicine, Stanford University, CA, USA. Electronic address: [email protected]. ... Results: Pfizer and Moderna mRNA COVID-19 vaccines were associated with an excess risk of serious adverse events of special interest of 10.1 and 15.1 per 10,000 vaccinated over placebo baselines of 17.6 and ...
By the beginning of June 2021, almost 11% of the world's population had received at least one dose of a coronavirus disease 2019 (COVID‐19) vaccine. 1 This represents an extraordinary scientific and logistic achievement — in 18 months, researchers, manufacturers and governments collaborated to produce and distribute vaccines that appear ...
A comprehensive research paper titled "Tracking Anti Vaccination Sentiment in Eastern European Social Media Networks" by UNICEF in 2013 found that vaccine influencers (people or pages that speak publicly about vaccines, both positively and negatively) are most prominent on Facebook and Twitter. This study found that Twitter users are ...
October 8, 2021. States have mandated vaccinations since long before COVID-19. By. Drew DeSilver. Students at Huntington Park High School in California wait in line to submit proof of immunization. (Irfan Khan/Los Angeles Times via Getty Images) Many Republican governors reacted furiously after President Joe Biden said he would require ...
During the COVID-19 pandemic, excess deaths including cancer have become a concern in Japan, which has a rapidly aging population. Thus, this study aimed to evaluate how age-adjusted mortality rates (AMRs) for different types of cancer in Japan changed during the COVID-19 pandemic (2020-2022). Official statistics from Japan were used to compare observed annual and monthly AMRs with predicted ...
Consequently, work on vaccines against SARS-CoV ended and its funding was cut. Only a whole inactivated vaccine 23 and a DNA vaccine 24 were tested in phase 1 clinical trials. Following a ...
University of California - Riverside. "Vaccine breakthrough means no more chasing strains." ScienceDaily. ScienceDaily, 15 April 2024. <www.sciencedaily.com / releases / 2024 / 04 / 240415163733 ...
April 18, 2024 10:36 AM PT. Scripps Research in La Jolla says it has taken a promising step toward developing a vaccine to fight the effects of xylazine, an animal tranquilizer that's illicitly ...
More than 3.5 million cases of dengue have been confirmed by governments in Latin America in the first three months of 2024, compared with 4.5 million in all of 2023. There have been more than ...
Introduction "The impact of vaccination on the health of the world's peoples is hard to exaggerate. With the exception of safe water, no other modality has had such a major effect on mortality reduction and population growth" (Plotkin and Mortimer, 1988).The development of safe and efficacious vaccination against diseases that cause substantial morbidity and mortality has been one of the ...
Vaccines are a clinical product that is composed of live or dead material from an infectious agent - bacterium, virus, fungus or parasite - that elicit protective immunity against the pathogen ...
How Pew Research Center will report on generations moving forward. Journalists, researchers and the public often look at society through the lens of generation, using terms like Millennial or Gen Z to describe groups of similarly aged people. This approach can help readers see themselves in the data and assess where we are and where we're ...
Antiretroviral Drugs for HIV Treatment and Prevention in Adults - 2022 IAS-USA Recommendations CONSERVE 2021 Guidelines for Reporting Trials Modified for the COVID-19 Pandemic Creation and Adoption of Large Language Models in Medicine Global Burden of Cancer, 2010-2019 Global Burden of Long COVID Global Burden of Melanoma Global Burden of Skin ...