
An official website of the United States government
The .gov means it’s official. Federal government websites often end in .gov or .mil. Before sharing sensitive information, make sure you’re on a federal government site.
The site is secure. The https:// ensures that you are connecting to the official website and that any information you provide is encrypted and transmitted securely.
- Publications
- Account settings
Preview improvements coming to the PMC website in October 2024. Learn More or Try it out now .
- Advanced Search
- Journal List
- Springer Nature - PMC COVID-19 Collection

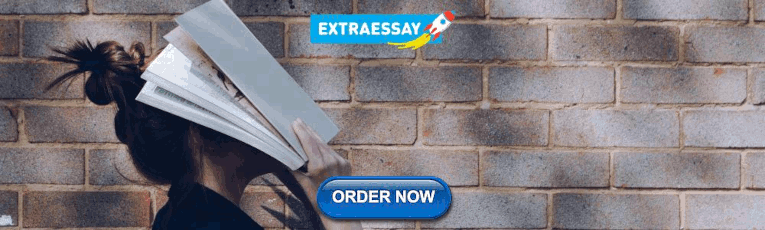
Climate change and its impact on biodiversity and human welfare
K. r. shivanna.
Ashoka Trust for Research in Ecology and the Environment, Srirampura, Jakkur Post, Bengaluru, 560064 India
Climate change refers to the long-term changes in temperature and weather due to human activities. Increase in average global temperature and extreme and unpredictable weather are the most common manifestations of climate change. In recent years, it has acquired the importance of global emergency and affecting not only the wellbeing of humans but also the sustainability of other lifeforms. Enormous increase in the emission of greenhouse gases (CO 2 , methane and nitrous oxide) in recent decades largely due to burning of coal and fossil fuels, and deforestation are the main drivers of climate change. Marked increase in the frequency and intensity of natural disasters, rise in sea level, decrease in crop productivity and loss of biodiversity are the main consequences of climate change. Obvious mitigation measures include significant reduction in the emission of greenhouse gases and increase in the forest cover of the landmass. Conference of Parties (COP 21), held in Paris in 2015 adapted, as a legally binding treaty, to limit global warming to well below 2 °C, preferably to 1.5 °C by 2100, compared to pre-industrial levels. However, under the present emission scenario, the world is heading for a 3–4 °C warming by the end of the century. This was discussed further in COP 26 held in Glasgow in November 2021; many countries pledged to reach net zero carbon emission by 2050 and to end deforestation, essential requirements to keep 1.5 °C target. However, even with implementation of these pledges, the rise is expected to be around 2.4 °C. Additional measures are urgently needed to realize the goal of limiting temperature rise to 1.5 °C and to sustain biodiversity and human welfare.
Introduction
Climate change refers to long-term changes in local, global or regional temperature and weather due to human activities. For 1000s of years, the relationship between lifeforms and the weather have been in a delicate balance conducive for the existence of all lifeforms on this Planet. After the industrial revolution (1850) this balance is gradually changing and the change has become apparent from the middle of the twentieth century. Now it has become a major threat to the wellbeing of humans and the sustainability of biodiversity. An increase in average global temperature, and extreme and unpredictable weather are the most common manifestations of climate change. It has now acquired the importance of global emergency. According to the report of the latest Intergovernmental Panel for Climate Change (AR6 Climate Change 2021 ), human-induced climate change as is prevalent now is unprecedented at least in the last 2000 years and is intensifying in every region across the globe. In this review the drivers of climate change, its impact on human wellbeing and biodiversity, and mitigation measures being taken at global level are briefly discussed.
Drivers of climate change
Emission of green-house gases.
Steady increase in the emission of greenhouse gases (GHGs) due to human activities has been the primary driver for climate change. The principal greenhouse gases are carbon dioxide (76%), methane (16%), and to a limited extent nitrous oxide (2%). Until recent decades, the temperature of the atmosphere was maintained within a reasonable range as some of the sunlight that hits the earth was reflected back into the space while the rest becomes heat that keeps the earth and the atmosphere warm enough for the sustenance of life forms. Accumulation of greenhouse gases combine with water vapour to form a transparent layer in the atmosphere that traps infrared radiation (net heat energy) emitted from the Earth’s surface and reradiates it back to Earth’s surface, thus contributing to the increasing temperature (greenhouse effect). Methane is 25 times and nitrous oxide 300 times more potent than CO 2 in trapping heat. Until 2019, the US, UK, European Union, Canada, Australia, Japan and Russia were the major CO 2 producers and were responsible for 61% of world’s emissions. Now, China produces the maximum amount of CO 2 (27%) followed by USA (11%) and India (6.6%); on per capita basis, however, India stands ninth.
The emission of GHGs is largely due to the burning of fossil fuels (coal, oil and natural gas) for automobiles and industries which result in carbon emissions during their extraction as well as consumption. The amount of CO 2 in the atmosphere before the industrial revolution used to be around 280 ppm and now it has increased to 412 ppm (as of 2019). Increase in the atmospheric temperature also leads to an increase in the temperature of the ocean. The oceans play an important role in the global carbon cycle and remove about 25% of the carbon dioxide emitted by human activities. Further, some CO 2 dissolves in the ocean water releasing carbonic acid which increases the acidity of the sea water. Rising ocean temperatures and acidification not only reduce their capacity to act as carbon sinks but also affect ocean ecosystems and the populations that relay on them.
Increasing demand for meat and milk has led to a significant increase in the population of livestock and conversion of enormous amount of the land to pasture and farm land to raise livestock. Ruminant animals (largely cows, buffaloes and sheep) produce large amounts of methane when they digest food (through enteric fermentation by microbes), adding to the greenhouse gases in the atmosphere (Sejiyan et al. 2016 ). To produce 1 kg of meat it requires 7 kg of grain and between 5000 and 20,000 L of water whereas to produce 1 kg of wheat it requires between 500 and 4000 L of water (Pimentel and Pimentel 2003 ). Anaerobic fermentation of livestock manure also produces methane. According to Patrick Brown, our animal farming industry needs to be changed; using readily available plant ingredients, the nutritional value of any type of meat can be matched with about one twentieth of the cost (See Leeming 2021 ).
The main natural source of nitrous oxide released to the atmosphere (60%) comes from the activity of microbes on nitrogen-based organic material from uncultivated soil and waste water. The remaining nitrous oxide comes from human activities, particularly agriculture. Application of nitrogenous fertilizers to crop plants is a routine practice to increase the yield; many of the farmers tend to apply more than the required amount. However, it results in nitrous oxide emissions from the soil through nitrification and denitrification processes by microbes. Both synthetic and organic fertilizers increase the amount of nitrogen available in the soil to microbial action leading to the release of nitrous oxide. Organic fertilizers, however, release nitrogen more slowly than synthetic ones so that most of it gets absorbed by the plants as they become available. Synthetic fertilizers release nitrogen rapidly which cannot be used by plants right away, thus making the excess nitrogen available to microbes to convert to nitrous oxide. Presently CO 2 concentration in the atmosphere is higher than at any time in at least 2 million years, and methane and nitrous oxide are higher than at any time in the last 800,000 years (AR6 Climate Change 2021 ).
Permafrost (permanently frozen soil), widespread in Arctic regions of Siberia, Canada, Greenland, Alaska, and Tibetan plateau contains large quantities of organic carbon in the top soil leftover from dead plants that could not be decomposed or rot away due to the cold. Global warming-induced thawing of permafrost facilitates decomposition of this material by microbes thus releasing additional amount of carbon dioxide and methane to the atmosphere.
Deforestation
Limited deforestation in early part of human civilization was the result of subsistence farming; farmers used to cut down trees to grow crops for consumption of their families and local population. In preindustrial period also, there was a balance between the amount of CO 2 emitted through various processes and the amount absorbed by the plants. Forests are the main sinks of atmospheric CO 2 . After the industrial revolution, the trend began to change; increasing proportion of deforestation is being driven by the demands of urbanization, industrial activities and large-scale agriculture. A new satellite map has indicated that field crops have been extended to one million additional km 2 of land over the last two decades and about half of this newly extended land has replaced forests and other ecosystems (Potapov et al. 2021 ).
In recent decades the demands on forest to grow plantation crops such as oil palm, coffee, tea and rubber, and for cattle ranching and mining have increased enormously thus reducing the forest cover. According to the World Wildlife Fund (WWF), over 43 million hectares of forest was lost between 2004 and 2017 out of 377 million hectares monitored around the world (Pacheco et al. 2021 ). Amazon Rain Forest is the largest tropical rain forest of the world and covers over 5 million km 2 . It is undergoing extensive degradation and has reached its highest point in recent years. According to National Geographic, about 17% of Amazon rain forest has been destroyed over the past 50 years and is increasing in recent years; during the last 1 year it has lost over 10,000 km 2 . In most of the countries the forest cover is less than 33%, considered necessary. For example, India’s forest and tree cover is only about 24.56% of the geographical area (Indian State Forest Report 2019 ).
Impacts of climate change
Increase in atmospheric temperature has serious consequences on biodiversity and ecosystems, and human wellbeing. The most important evidences of climate change is the long term data available on the CO 2 levels, global temperature and weather patterns. The impacts of climate change in the coming decades are based on published models on the basis of the analysis of the available data. Comparison of the performance of climate models published between 1970 and 2007 in projecting global mean surface temperature and associated changes with actual observations have shown that the models were consistent in predicting global warming in the years after publication (Hausfather et al. 2019 ). This correlation between predicted models and actual data indicates that the models are indeed reliable in accurately predicting the global warming and its impacts on weather pattern in the coming decades and their consequences on biodiversity and human welfare.
Weather pattern and natural disasters
One of the obvious changes observed in recent years is the extreme and unpredictable weather, and an increase in the frequency and intensity of natural disasters. Brazil’s south central region saw one of the worst droughts in 2021with the result many major reservoirs reached < 20% capacity, seriously affecting farming and energy generation (Getirana et al. 2021 ). In earlier decades, it was possible to predict with reasonable certainty annual weather pattern including the beginning and ending of monsoon rains; farmers could plan sowing periods of their crops in synchrony with the prevailing weather. Now the weather pattern is changing almost every year and the farmers are suffering huge losses. Similarly the extent of annual rainfall and the locations associated with heavy and scanty rainfall are no more predictable with certainty. Many areas which were associated with scanty rainfall have started getting much heavier rains and the extent of rainfall is getting reduced in areas traditionally associated with heavy rainfall. Similarly the period and the extent of snowfall in temperate regions have also become highly variable.
Increase in the frequency and intensity of natural disasters such as floods and droughts, cyclones, hurricanes and typhoons, and wildfires have become very obvious. Top five countries affected by climate change in 2021 include Japan, Philippines, Germany, Madagascar and India. Apart from causing death of a large number of humans and other animals, economic losses suffered by both urban and rural populations have been enormous. Deadly floods and landslides during 2020 forced about 12 million people leave their homes in India, Nepal and Bangladesh. According to World Meteorological Organization’s comprehensive report published in August 2021 (WMO-No.1267), climate change related disasters have increased by a factor of five over the last 50 years; however, the number of deaths and economic losses were reduced to 2 million and US$ 3.64 trillion respectively, due to improved warning and disaster management. More than 91% of these deaths happened in developing countries. Largest human losses were brought about by droughts, storms, floods and extreme temperatures. The report highlights that the number of weather, climate and water-extremes will become more frequent and severe as a result of climate change.
Global warming enhances the drying of organic matter in forests, thus increasing the risks of wildfires. Wildfires have become very common in recent years, particularly is some countries such as Western United States, Southern Europe and Australia, and are becoming more frequent and widespread. They have become frequent in India also and a large number of them have been recorded in several states. According to European Space Agency, fire affected an estimated four million km 2 of Earth’s land each year. Wildfires also release large amounts of carbon dioxide, carbon monoxide, and fine particulate matter into the atmosphere causing air pollution and consequent health problems. In 2021, wildfires around the world, emitted 1.76 billion tonnes of carbon (European Union’s Copernicus Atmospheric Monitoring Service). In Australia, more than a billion native animals reported to have been killed during 2020 fires, and some species and ecosystems may never recover (OXFAM International 2021 ).
Sea level rise
Global warming is causing mean sea level to rise in two ways. On one hand, the melting of the glaciers, the polar ice cap and the Atlantic ice shelf are adding water to the ocean and on the other hand the volume of the ocean is expanding as the water warms. Incomplete combustion of fossil fuels, biofuels and biomass releases tiny particles of carbon (< 2.5 µm), referred to as black carbon. While suspended in the air (before they settle down on earth’s surface) black carbon particles absorb sun’s heat 1000s of times more effectively than CO 2 thus contributing to global warming. When black particles get deposited over snow, glaciers or ice caps, they enhance their melting further adding to the rise in sea level. Global mean sea level has risen faster since 1900 than over any preceding century in at least the last 3000 years. Between 2006 and 2016, the rate of sea-level rise was 2.5 times faster than it was for almost the whole of the twentieth century (OXFAM International 2021 ). Precise data gathered from satellite radar measurements reveal an accelerating rise of 7.5 cm from 1993 to 2017, an average of 31 mm per decade (WCRP Global Sea Level Budget Group 2018 ).
Snow accounts for almost all current precipitation in the Arctic region. However, it continues to warm four times faster than the rest of the world as the melting ice uncovers darker land or ocean beneath, which absorbs more sunlight causing more heating. The latest projections indicate more rapid warming and sea ice loss in the Arctic region by the end of the century than predicted in previous projections (McCrystall et al. 2021 ). It also indicates that the transition from snow to rain-dominated Arctic in the summer and autumn is likely to occur decades earlier than estimated. In fact this transition has already begun; rain fell at Greenland’s highest summit (3216 m) on 14 August 2021 for several hours for the first time on record and air temperature remained above freezing for about 9 h (National Snow and Ice Sheet Centre Today, August 18, 2021).
In the annual meeting of the American Geophysical Union (13 December 2021) researchers warned that rapid melting and deterioration of one of western Antarctica’s biggest glaciers, roughly the size of Florida, Thwaites (often called as Doomsday Glacier), could lead to ice shelf’s complete collapse in just a few years. It holds enough water to raise sea level over 65 cm. Thwaites glacier is holding the entire West Antarctic ice sheet and is being undermined from underneath by warm water linked to the climate change. Melting of Thwaites could eventually lead to the loss of the entire West Antarctic Ice Sheet, which locks up 3.3 m of global sea level rise. Such doomsday may be coming sooner than expected (see Voosen 2021 ). If this happens, its consequences on human tragedy and biodiversity loss are beyond imagination.
The Himalayan mountain range is considered to hold the world’s third largest amount of glacier ice after Arctic and Antarctic regions. It is considered as Asian water tower (Immerzeel et al. 2020 ); the meltwater from the Himalayan glaciers provide the source of fresh water to nearly 2 billion people living along the mountain valleys and lowlands around the Himalayas. These glaciers are melting at unprecedented rates. Recently King et al. ( 2021 ) studied 79 glaciers close to Mt. Everest by analysing mass-change measurements from satellite archives and reported that the rate of ice loss from glaciers consistently increased since the early 1960s. This loss is likely to increase in the coming years due to further warming. In another study, a tenfold acceleration in ice loss was observed across the Himalayas than the average rate in recent decades over the past centuries (Lee et al. 2021 ). Melting of glaciers also results in drying up of perennial rivers in summer leading to the water scarcity for billions of humans and animals, and food and energy production downstream. See level rise and melting of glaciers feeding the rivers could lead to migration of huge population, creating additional problems. Even when the increase in global temperature rise is limited to 1.5 °C (discussed later), it generates a global sea-level rise between 1.7 and 3.2 feet by 2100. If it increases to 2 °C, the result could be more catastrophic leading to the submergence of a large number of islands, and flooding and submergence of vast coastal areas, saltwater intrusion into surface waters and groundwater, and increased soil erosion. A number of islands of Maldives for example, would get submerged as 80% of its land area is located less than one meter above the sea level. The biodiversity in such islands and coastal areas becomes extinct. China, Vietnam, Fiji, Japan, Indonesia, India and Bangladesh are considered to be the most at risk. Sundarbans National Park (UNESCO world heritage Site), the world’s largest Mangrove Forest spread over 140,000 hectares across India and Bangladesh, is the habitat for Royal Bengal Tiger and several other animal species. The area has already lost 12% of its shoreline in the last four decades by rising see level; it is likely to be completely submerged. Jakarta in Indonesia is the fastest sinking city in the world; the city has already sunk 2.5 m in the last 10 years and by 2050, most of it would be submerged. In Europe also, about three quarters of all cities will be affected by rising sea levels, especially in the Netherlands, Spain, Belgium, Greece and Italy. The entire city of Venice may get submerged (Anonymous 2018 ). In USA, New York City and Miami would be particularly vulnerable.
Crop productivity and human health
Many studies have indicated that climate change is driving increasing losses in crop productivity (Zhu et al. 2021 ). The models on global yield loss for wheat, maize and rice indicate an increase in yield losses by 10 to 25% per degree Celsius warming (Deutsch et al. 2018 ). Bras et al. ( 2021 ) reported that heatwave and drought roughly tripled crop losses over the last 50 years, from − 2.2% (1964–1990) to − 7.3% (1991–2015). Overall, the loss in crop production from climate-driven abiotic stresses may exceed US$ 170 billion year –1 and represents a major threat to global food security (Razaaq et al. 2021 ). Analysis of annual field trials of common wheat in California from 1985 to 2019 (35 years), during which the global atmospheric CO 2 concentration increased by 19%, revealed that the yield declined by 13% (Bloom and Plant 2021 ). Apart from crop yield, climate change is reported to result in the decline of nutritional value of food grains (Jagermeyr et al. 2021 ). For example, rising atmospheric CO 2 concentration reduces the amounts of proteins, minerals and vitamins in rice (Zhu et al. 2018 ). This may be true in other cereal crops also. As rice supplies 25% of all global calories, this would greatly affect the food and nutritional security of predominantly rice growing countries. Climate change would also increase the prevalence of insect pests adding to the yield loss of crops. The prevailing floods and droughts also affect food production significantly. Global warming also affects crop productivity through its impact on pollinators. Insect pollinators contribute to crop production in 75% of the leading food crops (Rader et al. 2013 ). Climate change contributes significantly to the decline in density and diversity of pollinators (Shivanna 2020 ; Shivanna et al. 2020 ). Under high as well as low temperatures, bees spend less time in foraging (Heinrich 1979 ) adding additional constraints to pollination efficiency of crop species.
The IPCC Third Assessment Report (Climate change 2001: The scientific basis – IPCC) concluded that the poorest countries would be hardest hit with reductions in crop yields in most tropical and sub-tropical regions due to increased temperature, decreased water availability and new or changed insect pest incidence. Rising ocean temperatures and ocean acidification affect marine ecosystems. Loss of fish habitats is modifying the distribution and productivity of both marine and freshwater species thus affecting the sustainability of fisheries and populations dependent on them (Salvatteci 2022 ).
Air pollution is considered as the major environmental risk of climate change due to its impact on public health causing increasing morbidity and mortality (Manisalidis 2020 ). Particulate matter, carbon monoxide, nitrogen oxide, and sulphur dioxide are the major air pollutants. They cause respiratory problems such as asthma and bronchiolitis and lung cancer. Recent studies have indicated that exposure to air pollution is linked to methylation of immunoregulatory genes, altered immune cell profiles and increased blood pressure in children (Prunicki et al. 2021 ). In another study wildfire smoke has been reported to be more harmful to humans than automobiles emissions (Aquilera et al. 2021 ). Stubble burning (intentional incineration of stubbles by farmers after crop harvest) has been a common practice in some parts of South Asia particularly in India; it releases large amount of toxic gases such as carbon monoxide and methane and causes serious damage to the environment and health (Abdurrahman et al. 2020 ). It also affects soil fertility by destroying the nutrients and microbes of the soil. Attempts are being made to use alternative methods to prevent this practice.
A number of diseases such as zika fever, dengue and chikungunya are transmitted by Aedes mosquitoes and are now largely restricted to the monsoon season. Global warming facilitates their spread in time and space thus exposing new populations and regions for extended period to these diseases. Lyme disease caused by a bacterium is transmitted through the bite of the infected blacklegged ticks. It is one of the most common disease in the US. The cases of Lyme disease have tripled in the past two decades. Recent studies have suggested that variable winter conditions due to climate change could increase tick’s activity thus increasing the infections (see Pennisi 2022 ).
Biodiversity
Biodiversity and associated ecoservices are the basic requirements for human livelihood and for maintenance of ecological balance in Nature. Documentation of biodiversity, and its accelerating loss and urgent need for its conservation have become the main concern for humanity since several decades (Wilson and Peter 1988 ; Wilson 2016 ; Heywood 2017 ; IPBES 2019 ; Genes and Dirzo 2021 ; Shivanna and Sanjappa 2021 ). It is difficult to analyse the loss of biodiversity exclusively due to climate change as other human-induced environmental changes such as habitat loss and degradation, overexploitation of bioresources and introduction of alien species also interact with climate change and affect biodiversity and ecosystems. In recent decades there has been a massive loss of biodiversity leading to initiation of the sixth mass extinction crisis due to human-induced environmental changes. These details are not discussed here; they are dealt in detail in many other reviews (Leech and Crick 2007 ; Sodhi and Ehrlich 2010 ; Lenzen et al. 2012 ; Dirzo and Raven 2003 ; Raven 2020 ; Ceballos et al. 2015 ; Beckman et al. 2020 ; Shivanna 2020 ; Negrutiu et al. 2020 ; Soroye et al. 2020 ; Wagner 2020 , 2021 ; Anonymous 2021 ; Zattara and Aizen 2021 ).
Terrestrial species
There are several effects on biodiversity caused largely by climate change. Maxwell et al. ( 2019 ) reviewed 519 studies on ecological responses to extreme climate events (cyclones, droughts, floods, cold waves and heat waves) between 1941 and 2015 covering amphibians, birds, fish, invertebrates, mammals, reptiles and plants. Negative ecological responses have been reported for 57% of all documented groups including 31 cases of local extirpations and 25% of population decline.
Increase in temperature impacts two aspects of growth and development in plants and animals. One of them is a shift in distributional range of species and the other is the shift in phenological events. Plant and animal species have adapted to their native habitat over 1000s of years. As the temperature gets warmer in their native habitat, species tend to move to higher altitudes and towards the poles in search of suitable temperature and other environmental conditions. There are a number of reports on climate change-induced shifts in the distributional range of both plant and animal species (Grabherr et al. 1994 ; Cleland et al. 2007 ; Parmesan and Yohe 2003 ; Beckage et al. 2008 ; Pimm 2009 ; Miller-Rushing et al. 2010 ; Lovejoy and Hannah 2005 ; Lobell et al. 2011 ). Many species may not be able to keep pace with the changing weather conditions and thus lag behind leading to their eventual extinction. Long-term observations extending for over 100 years have shown that many species of bumblebees in North-America and Europe are not keeping up with the changing climate and are disappearing from the southern portions of their range (Kerr et al. 2015 ). Most of the flowering plants depend on animals for seed dispersal (Beckman et al. 2020 ). Defaunation induced by climate change and other environmental disturbances has reduced long-distance seed dispersal. Prediction of dispersal function for fleshly-fruited species has already reduced the capacity of plants to track climate change by 60%, thus severely affecting their range shifts (Fricke et al. 2022 ).
Climate change induced shifts in species would threaten their sustenance even in protected areas as they hold a large number of species with small distributional range (Velasquez-Tibata et al. 2013 ). Pautasso ( 2012 ) has highlighted the sensitivity of European birds to the impacts of climate change in their phenology (breeding time), migration patterns, species distribution and abundance. Metasequoia glyptostroboides is one of the critically endangered species with extremely small populations distributed in South-Central China. Zhao et al. ( 2020 ) analysed detailed meteorological and phenological data from 1960 to 2016 and confirmed that climate warming has altered the phenology and compressed the climatically suitable habitat of this species. Their studies revealed that the temperature during the last 57 years has increased significantly with the expansion of the length of growing season of this species. Climatically suitable area of the species has contracted at the rate of 370.8 km 2 per decade and the lower and upper elevation limits shrunk by 27 m over the last 57 years.
The other impact of climate change on plant and animal species has been in their phenological shift. Phenology is the timing of recurring seasonal events; it is a sort of Nature’s calendar for plants and animals. In flowering plants, various reproductive events such as the timing of flowering, fruiting, their intensity, and longevity are important phenological events, and in animals some of the phenological events include building of nests in birds, migration of animal species, timing of egg laying and development of the larva, pupa and adult in insects. Phenological events of both plants and animals are generally fixed in specific time of the year as they are based on environmental cues such as temperature, light, precipitation and snow melt. Phenological timings of species are the results of adaptations over 100 s of years to the prevailing environment. Wherever there is a mutualism between plants and animals, there is a synchrony between the two partners. For example in flowering plants, flowering is associated with the availability of pollinators and fruiting is associated with the availability of seed dispersers and optimal conditions for seed germination and seedling establishment. In animals also, phenological events are adapted to suit normal growth and reproduction. In temperate regions, melting of ice initiates leafing in plants; this is followed by the flowering in the spring. Similarly, warming of the climate before the spring induces hatching of the hibernating insects which feed on newly developed foliage. Insects emerge and ready to pollinate the flowers by the time the plants bloom.
The dates of celebration of the cherry blossom festival, an important cultural event in Japan that coincides with the peak of flowering period of this species and for which > 1000 years of historical records are available, has shown advances in the dates of the festival in recent decades (Primack et al. 2009 ). The records between 1971 and 2000 showed that the trees flowered an average of 7 days earlier than all the earlier years (Allen et al. 2013 ). These advances were correlated with increasing temperature over the years. Spring temperatures in the Red River valley, North Dakota, USA have extended the period of the growing season of plants significantly over the years. Flowering times, for which data are available from1910 to1961, have been shown to be sensitive to at least one variable related to temperature or precipitation for 75% of the 178 species investigated (Dunnell and Traverse 2011 ). The first flowering time has been significantly shifted earlier or later over the last 4 years of their study in 5–15% of the observed species relative to the previous century. Rhododendron arboretum , one of the central Himalayan tree species, flowers from early February to mid-March. Generalized additive model using real-time field observations (2009–2011) and herbarium records (1893–2003) indicated 88–97 days of early flowering in this species over the last 100 years (Gaira et al. 2014 ). This early flowering was correlated with an increase in the temperature.
One of the consequences of a shift in the distributional range of species and phenological timings is the possible uncoupling of synchronization between the time of flowering of plant species and availability of its pollinators (see Gerard et al. 2020 ). When a plant species migrates, its pollinator may not be able to migrate; similarly when a pollinator migrates, the plant species on which it depends for sustenance may not migrate. Memmott et al. ( 2007 ) explored potential disruption of pollination services due to climate change using a network of 1420 pollinators and 429 plant species by simulating consequences of phenological shifts that can be expected with doubling of atmospheric CO 2 . They reported phenological shifts which reduced available floral resources to 17–50% of all pollinator species. A long-term study since the mid-1970s in the Mediterranean Basin has indicated that unlike the synchrony present in the earlier decades between the flowering of plant species and their pollinators, insect phenoevents during the last decade showed a steeper advance than those of plants (Gordo and Sanz 2005 ). Similar asynchrony has been reported between the flowering of Lathyrus and one of its pollinators, Hoplitis fulgida (Forrest and Thomson 2011 ). Asynchrony between flowering and appearance of pollinator has also been reported in a few other cases (Kudo and Ida 2013 ; Kudo 2014 ). Such asynchrony could affect the sustenance of plant and/or pollinator species in the new environment.
Marine species
Amongst the marine species, corals are the most affected groups due to the rise in temperature and acidity of oceans. Corals live in a symbiotic relationship with algae which provide colour and photosynthates to the corals. Corals are extremely sensitive to heat and acidity; even an increase of 2–3°F of ocean water above normal results in expulsion of the symbiotic algae from their tissues leading to their bleaching (Hoegh-Guldberg et al. 2017 ). When this bleached condition continues for several weeks, corals die. Nearly one-third of the Great Barrier Reef, the world’s largest coral reef system that sustains huge Australian tourism industry, has died as a result of global warming (Hughes et al. 2018 ). According to the experts the reef will be unrecognizable in another 50 years if greenhouse gas emissions continue at the current rate.
According to UNESCO, coral reefs in all 29 reef-containing World Heritage sites would cease to exist as functioning ecosystems by the end of this century if greenhouse gas emissions continue to be emitted at the present rate (Elena et al. 2020 ). Recent assessment of the risk of ecosystem collapse to coral reefs of the Western Indian Ocean, covering about 5% of the global total, range from critically endangered to vulnerable (Obura et al. 2021 ). Coral reefs provide suitable habitat for thousands of other species, including sharks, turtles and whales. If corals die, the whole ecosystem will get disrupted.
Melting of ice in Arctic region due to global warming is threatening the survival of native animals such as polar bear, Arctic fox and Arctic wolf. Rising of sea level also leads to the extinction of a large number of endangered and endemic plant and animal species in submerged coastal areas and islands. Over 180,000 islands around the globe contain 20% of the world’s biodiversity. Bellard et al. ( 2013 ) assessed consequences of sea level rise of 1–6 m for 10 insular biodiversity hotspots and their endemic species at the risk of potential extinction. Their study revealed that 6 to19% of the 4447 islands would be entirely submerged depending on the rise of sea level; three of them, the Caribbean islands, the Philippines and Sundaland, displayed the most significant hotspots representing a potential threat for 300 endemic species. According to the Centre for Biological Diversity ( 2013 ) 233 federally protected threatened and endangered species in 23 coastal states are threatened if rising sea is unchecked. Recently more than 100 Aquatic Science Societies representing over 80,000 scientists from seven continents sounded climate alarm (Bonar 2021 ). They have highlighted the effects of climate change on marine and aquatic ecosystems and have called on the world leaders and public to undertake mitigation measures to protect and sustain aquatic systems and theirs services.
Mitigation measures
The principal mitigation measures against climate change are obvious; they include significant reduction in greenhouse gas emission, prevention of deforestation and increase in the forest cover. To reduce greenhouse gas emission, use of coal and fossil fuels needs to be reduced markedly. As climate change is a global challenge, local solutions confined to one or a few countries do not work; we need global efforts. Many attempts are being made to achieve these objectives at the global level since many decades. Mitigation measures are largely at the level of diplomatic negotiations involving states and international organizations, Governments and some nongovernmental organizations. The Intergovernmental Panel on Climate Change (IPCC) was established by the United Nations Environment Programme (UNEP) and the World Meteorological Organization (WMO) in 1988. Its mandate was to provide political leaders with periodic scientific assessments concerning climate change, its implications and risks, and also to put forward adaptation and mitigation strategies. In 1992 more than 1700 World scientists, including the majority of living Nobel laureates gave the first Warning to Humanity about climate change and associated problems. They expressed concern about potential damage to the Planet Earth by human-induced environmental changes such as climate change, continued human population growth, forest loss, biodiversity loss and ozone depletion. Conference of Parties (COP) of the UN Convention on Climate Change was established in 1992 under the United Nations Framework Convention on Climate Change (UNFCCC) to discuss global response to climate change. Its first meeting (COP 1) was held in Berlin in March 1995 and is being held every year since then. The Fifth Assessment Report of the IPCC, released in November 2014, projected an increase in the mean global temperature of 3.7 to 4.8 °C by 2100, relative to preindustrial levels (1850), in the absence of new policies to mitigate climate change; it highlighted that such an increase would have serious consequences. This prediction compelled the participating countries at the COP 21 held in Paris in December 2015 to negotiate effective ways and means of reducing carbon emissions. In this meeting the goal to limit global warming to well below 2 °C, preferably to 1.5 °C, compared to preindustrial levels was adapted by 196 participating countries as a legally binding treaty on climate change. It also mandated review of progress every 5 years and the development of a fund containing $100 billion by 2020, which would be replenished annually, to help developing countries to adopt non-greenhouse-gas-producing technologies.
In 2017, after 25 years after the first warning, 15,354 world scientists from 184 countries gave ‘second warning to humanity’ (Ripple et al. 2017 ). They emphasized that with the exception of stabilizing the stratospheric ozone layer, humanity has failed to make sufficient progress in solving these environmental challenges, and alarmingly, most of them are getting far worse. Analysis of Warren et al. ( 2018 ) on a global scale on the effects of climate change on the distribution of insects, vertebrates and plants indicated that even with 2 °C temperature increase, approximately 18% of insects, 16% of plants and 8% of vertebrates species are projected to loose > 50% geographic range; this falls to 6% for insects, 8% for plants and 4% for vertebrates when temperature increase is reduced to 1.5 °C.
UN Report on climate change (prepared by > 90 authors from 40 countries after examining 6000 scientific publications) released in October 2018 in South Korea also gave serious warning to the world. Some of the salient features of this report were:
- Overshooting 1.5 °C will be disastrous. It will have devastating effects on ecosystems, communities and economies. By 2040 there could be global food shortages, the inundation of coastal cities and a refugee crisis unlike the world has ever seen.
- Even 1.5 °C warming would rise sea levels by 26–77 cm by 2100; 2 °C would add another 10 cm which would affect another 10 million people living in coastal regions.
- Coral reefs are projected to decline 70–90% even at 1.5 °C. At 2 °C, 99% of the reefs would be ravaged.
- Storms, floods, droughts and forest fires would increase in intensity and frequency.
- The world has already warmed by about 1 °C since preindustrial times. We are currently heading for about 3–4 °C of warming by 2100.
- Unless rapid and deep reductions in CO 2 and other greenhouse gas emissions occur in the coming decades, achieving the goals of the 2015 Paris Agreement will be beyond reach.
- To keep 1.5 °C target, coal’s share of global electricity generation must be cut from the present 37% to no more than 2% by 2050. Renewable power must be greatly expanded. Net CO 2 emissions must come down by 45% (from 2010 levels) by 2030 and reach net zero (emissions of greenhouse gases no more than the amount removed from the atmosphere) around 2050.
This report awakened the world Governments about the seriousness of the climate change. The COP 26 meeting which was to be held in 2020 had to be postponed due to Covid-19 pandemic. The first part of the sixth report of IPCC was released in August 2021 (AR6 Climate Change 2021 ), just before the postponed COP 26 meeting was to be held; it highlighted that the threshold warming of 1.5 °C (the target of keeping the warming by the end of the century) would reach in the next 20 years itself and if the present trends continue, it would reach 2.7 °C by the end of the century.
Under this predicted climate emergency (see Ripple et al. 2020 ), COP 26 meeting was held in Glasgow, Scotland between October 31 and November 12, 2021. Nearly 200 countries participated in this meeting. The main aim of the COP 26 was finalization of the rules and procedures for implementation of the Paris agreement to keep the temperature increase to 1.5 °C. A number of countries including USA and European Union pledged to reach net zero carbon emission by 2050. China pledged to reach net zero emissions by 2060 and India by 2070. India also committed to reduce the use of fossil fuels by 40% by 2030. More than 100 countries committed to reduce worldwide methane emissions by 30% (of 2020 levels) by 2030 and to end deforestation by 2030. The average atmospheric concentration of methane reached a record 1900 ppb in September 2021; it was 1638 ppb in 1983 (US National Oceanic and Atmospheric Administration), highlighting the importance of acting on pledges made at the COP 26.
One of the limitations of COP meetings has been nonadherence of the commitment made by developed countries at Paris meeting to transfer US $100 billion annually to developing and poor countries to support climate mitigation and loss of damage, through 2025; only Germany, Norway and Sweden are paying their share. Several experts feel that the adoption of the Glasgow Climate Pact was weaker than expected. According to the assessment of Climate Action Tracker, a non-profit independent global analysis platform, emission reduction commitments by countries still lead to 2.4 °C warming by 2100. However, a positive outcome of the meeting was that it has kept alive the hopes of achieving the 1.5 °C goal by opening the options for further discussion in the coming COP meetings. Apart from implementation of mitigation pledges made by countries, it is also important to pay attention to climate adaptation since the negative effects of climate change will continue for decades or longer (AR6 Climate Change 2021 ). Investment in early warning is an important means of climate adaptation, which is lacking in many parts of Africa and Latin America.
Conclusions
Climate change has now become the fastest growing global threat to human welfare. The world has realized the responsibility of the present generation as it is considered to be the last generation capable of taking effective measures to reverse its impact. If it fails, human civilization is likely to be doomed beyond recovery. As emphasized by many organizations, the climate crisis is inherently unfair; poorer countries will suffer its consequences more than others. India is one amongst the nine countries identified to be seriously affected by climate change. According to a WHO analysis ( 2016 ) India could face more than 25% of all global climate-related deaths by 2050 due to decreasing food availability. China is expected to face the highest number of per capita food insecurity deaths. Bhutan, a small Himalayan kingdom with 60% forest cover, is the most net negative carbon emission country; its GHG emission is less than the amount removed from the atmosphere. Other countries should aim to emulate Bhutan as early as possible.
A number of other options have been suggested to trap atmospheric carbon dioxide (Climate change mitigation—Wikipedia). Carbon storage through sequestration of organic carbon by deep-rooted grasses has been one such approach (Fisher et al. 1994 ). Several studies from Africa have indicated that introduction of Brachiaria grasses in semi-arid tropics can help to increase not only carbon stock in the soil but also yield greater economic returns (Gichangi et al. 2017 ). Recently a new seed bank, ‘Future Seeds’ was dedicated at Palmira, Columbia to store world’s largest collection of beans, cassava, and tropical forage grasses for the use of breeders to create better performing and climate-resistant crops (Stokstad 2022 ). Brachiaria humidicola is one of the tropical forage grass stored in this seed bank for its potential benefit in carbon sequestration. Lavania and Lavania ( 2009 ) have suggested vetiver ( Vetiveria zizanioides ), a C 4 perennial grass, with massive fibrous root system that can grow up to 3 m into the soil in 1 year, as a potential species for this purpose. Vetiver is estimated to produce 20–30 tonnes of root dry matter per hectare annually and holds the potential of adding 1 kg atmospheric CO 2 annually to the soil carbon pool per m 2 surface area. Carbon dioxide capture and storage is another such potential approach. At present it is too expensive and this approach may have to wait until improvement of the technology, reduction in the cost and feasibility of transfer of the technology to developing countries (IPCC Special Report on carbon dioxide capture and storage 2005 ).
There has been some discussion on the role of climate change on speciation (Levin 2019 ; Gao et al. 2020 ). Some evolutionary biologists have observed that the rate of speciation has accelerated in the recent past due to climate change and would continue to increase in the coming decades (Thomas 2015 ; Levin 2019 ; Gao et al. 2020 ). They propose that auto- and allo-polyploidy are going to be the primary modes of speciation in the next 500 years (Levin 2019 , see also Gao 2019 , Villa et al. 2022 ). However, extinction of species imposed by climate change may excel positive impact on plant speciation via polyploidy (Gao et al. 2020 ). The question is will climate change induce higher level of polyploidy and other genetic changes in crop species also that would promote evolution of new genotypes to sustain productivity and quality of food grains? If so, it would ameliorate, to some extent, food and nutritional insecurity of humans especially in the developing world.
Effective implementation of the pledges made by different countries in COP 26 and actions to be taken in the coming COP meetings are going to be crucial and determine humanity’s success or failure in tackling climate change emergency. COP 26 climate pact to cut greenhouse gas emissions, end of deforestation and shift to sustainable transport is certainly more ambitious then earlier COPs. There are also many other positive signals for reducing fossil fuels. Scientists have started using more precise monitoring equipment to collect more reliable environmental data, and more options are being developed by researchers on renewable and alternate energy sources, and to capture carbon from industries or from the air (Chandler D, MIT News 24 Oct 2019, Swain F, BBC Future Planet, 12 March 2021). Scotland has become coal-free and Costa Rica has achieved 99% renewable energy. India has reduced the use of fossil fuel by 40% of it installed capacity, 8 years ahead of its commitment at the COP 26.
Further, people are becoming more conscious to reduce carbon emission by following climate-friendly technologies. Human sufferings associated with an increase in natural disasters throughout the world have focussed public attention on climate change as never before. They also realise the benefits of improved air quality by reducing consumption of coal and fossil fuels on health and ecosystems. The demand for electric vehicles is steadily growing. Reforestation is being carried out in a large scale in many countries. Recent studies across a range of tree plantations and native forests in 53 countries have revealed that carbon storage, soil erosion control, water conservation and biodiversity benefits are delivered better from native forests compared to monoculture tree plantations, although the latter yielded more wood (Hua et al. 2022 ). This has to be kept in mind in reforestation programmes. Hopefully the world will be able to realize the goal of limiting the temperature rise to 1.5 °C by the end of the century and humanity would learn to live in harmony with Nature.
Declarations
The author declares no conflict of interest.
- Abdurrahman MI, Chaki S, Saini G. Stubble burning: effects on health & environment, regulations and management practices. Environ. Adv. 2020 doi: 10.1016/j.envadv.2020.100011. [ CrossRef ] [ Google Scholar ]
- Allen JM, Terres MA, Katsuki T, et al. Modelling daily flowering probabilities: expected impact of climate change on Japanese cherry phenology. Global Change Biol. 2013; 20 :1251–1263. doi: 10.1111/gcb.12364. [ PubMed ] [ CrossRef ] [ Google Scholar ]
- Anonymous (2018) https://www.euronews.com/2018/02/02/rising-sea-levels-threat-a-shrinking-european-coastline-in-2100
- Anonymous (2021) Special Issue. Global decline in the Anthropocene. Proc Natl Acad Sci, USA 118: No 2
- Aquilera R, Curringham TW, Gershunov A, Benmarhnia T. Wildfire smoke impacts respiratory health more than fine particles from other sources: observational evidence from Southern California. Nat. Commun. 2021 doi: 10.1038/s41467-021-21708-0. [ PMC free article ] [ PubMed ] [ CrossRef ] [ Google Scholar ]
- AR6 Climate Change: The sixth assessment report on climate change. IPCC, Geneva (2021). https://www.ipcc.ch/report/ar6/wg1/
- Beckage B, Osborne B, Gavin DG, et al. A rapid upward shift of a forest ecotone during 40 years of warming in the Green Mountains of Vermont. Proc. Natl. Acad. Sci. USA. 2008; 105 :4197–4202. doi: 10.1073/pnas.0708921105. [ PMC free article ] [ PubMed ] [ CrossRef ] [ Google Scholar ]
- Beckman NG, Aslan CE, Rogers HS. The role of seed dispersal in plant populations: perspectives and advances in a changing world. AoB Plants. 2020 doi: 10.1093/aobpla/plaa010. [ PMC free article ] [ PubMed ] [ CrossRef ] [ Google Scholar ]
- Bellard C, Leclerc C, Courchamp F. Impact of sea level rise on the 10 insular biodiversity hotspots. Global Ecol. Biogeogr. 2013 doi: 10.1111/geb.12093. [ CrossRef ] [ Google Scholar ]
- Bloom AJ, Plant RC. Wheat grain yield decreased over the past 35 years, but protein content did not change. J. Exptl. Bot. 2021; 72 :6811–6821. doi: 10.1093/jxb/erab343. [ PubMed ] [ CrossRef ] [ Google Scholar ]
- Bonar SA. More than 111 aquatic-science societies sound climate alarm. Nature. 2021; 589 :352. doi: 10.1038/d41586-021-00107-x. [ PubMed ] [ CrossRef ] [ Google Scholar ]
- Bras TA, Seixas J, Nuno C, Jonas J. Severity of drought and heatwave crop losses tripled over the last five decades in Europe. Environ. Res. Lett. 2021 doi: 10.1088/1748-9326/abf004. [ CrossRef ] [ Google Scholar ]
- Ceballos G, Ehrlich PP, Barnosky AD, et al. Accelerated modern human-induced species losses: Entering the sixth mass extinction. Sci. Adv. 2015; 1 :e1400253. doi: 10.1126/sciadv.1400253. [ PMC free article ] [ PubMed ] [ CrossRef ] [ Google Scholar ]
- Centre for Biological Diversity: Deadly Waters: How Rising Seas Threaten 233 Endangered Species. (2013) https://www.biologicaldiversity.org/campaigns/sea-level_rise/pdfs/Sea_Level_Rise_Report_2013_web.pdf
- Cleland EE, Chuine I, Menzel A, et al. Shifting plant phenology in response to global change. Trends Ecol. Evol. 2007; 22 :357–365. doi: 10.1016/j.tree.2007.04.003. [ PubMed ] [ CrossRef ] [ Google Scholar ]
- Deutsch CA, Tewksbury JJ, Tigchelaar M, et al. Increase in crop losses to insect pests in a warming climate. Science. 2018; 361 :916–919. doi: 10.1126/science.aat3466. [ PubMed ] [ CrossRef ] [ Google Scholar ]
- Dirzo R, Raven PH. Global state of biodiversity and loss. Ann. Rev. Environ. Res. 2003; 28 :137–167. doi: 10.1146/annurev.energy.28.050302.105532. [ CrossRef ] [ Google Scholar ]
- Dunnell KL, Travers SE. Shifts in the flowering phenology of the northern Great Plains: patterns over 100 years. Am. J. Bot. 2011; 98 :935–945. doi: 10.3732/ajb.1000363. [ PubMed ] [ CrossRef ] [ Google Scholar ]
- Elena O, Matthew E-S, Matea O, et al. A conservation assessment of all natural World Heritage sites. Gland: IUCN; 2020. IUCN World Heritage Outlook 3. [ Google Scholar ]
- Fisher MJ, Rao IM, Ayarza MA, et al. Carbon storage by introduced deep-rooted grasses in the South American Savannas. Nature. 1994; 371 :236–238. doi: 10.1038/371236a0. [ CrossRef ] [ Google Scholar ]
- Forrest JRK, Thomson JD. An examination of synchrony between insect emergence and flowering in the Rocky Mountain meadows. Ecol. Monogr. 2011; 81 :469–491. doi: 10.1890/10-1885.1. [ CrossRef ] [ Google Scholar ]
- Fricke EC, Ordonez A, Rogers HS, et al. The effects of defaunation on plants’ capacity to track climate change. Science. 2022; 375 :210–214. doi: 10.1126/science.abk3510. [ PubMed ] [ CrossRef ] [ Google Scholar ]
- Gaira KS, Rawal R, Rawat B, Bhatt ID. Impact of climate change on the flowering of Rhododendron arboreum in central Himalaya, India. Curr. Sci. 2014; 106 :1735–1738. [ Google Scholar ]
- Gao JG. Dominant plant speciation types. A commentary on: plant speciation in the age of climate change. Ann. Bot. 2019; 124 :iv–vi. doi: 10.1093/aob/mcz174. [ PMC free article ] [ PubMed ] [ CrossRef ] [ Google Scholar ]
- Gao JG, Liu H, Wang N, et al. Plant extinction excels plant speciation in the Anthropocene. BMC Plant Biol. 2020; 20 :430. doi: 10.1186/s12870-020-02646-3. [ PMC free article ] [ PubMed ] [ CrossRef ] [ Google Scholar ]
- Genes L, Dirzo R. Restoration of plant–animal interactions in terrestrial ecosystems. Biol. Conserv. 2021 doi: 10.1016/j.biocon.2021.109393. [ CrossRef ] [ Google Scholar ]
- Gerard M, Vanderplanck M, Wood T, Michez D. Global warming and plant-pollinator mismatches. Emerg. Top. Life Sci. 2020; 4 :77–86. doi: 10.1042/ETLS20190139. [ PMC free article ] [ PubMed ] [ CrossRef ] [ Google Scholar ]
- Getirana A, Libonati R, Cataldi M. Brazil is in water crisis—it needs a drought plan. Nature. 2021; 600 :218–220. doi: 10.1038/d41586-021-03625-w. [ PubMed ] [ CrossRef ] [ Google Scholar ]
- Gichangi EM, Njarui DMG, Gatheru M. Plant shoots and roots biomass of Brachiaria grasses and their effect on soil carbon in the semi-arid tropics of Kenya. Trop. Subtrop. Agroecosyst. 2017; 20 :65–74. [ Google Scholar ]
- Gordo O, Sanz JJ. Phenology and climate change: a long-term study in a Mediterranean locality. Oecologia. 2005; 146 :484–495. doi: 10.1007/s00442-005-0240-z. [ PubMed ] [ CrossRef ] [ Google Scholar ]
- Grabherr G, Gottfried M, Pauli H. Climate effects on mountain plants. Nature. 1994; 369 :448. doi: 10.1038/369448a0. [ PubMed ] [ CrossRef ] [ Google Scholar ]
- Hausfather Z, Drake HF, Abbott T, Schmidt GA. Evaluating the performance of past climate model projections. Geophys. Res. Lett. 2019 doi: 10.1029/2019GL085378. [ CrossRef ] [ Google Scholar ]
- Heinrich B. Keeping a cool head: honeybee thermoregulation. Science. 1979; 205 :1269–1271. doi: 10.1126/science.205.4412.1269. [ PubMed ] [ CrossRef ] [ Google Scholar ]
- Heywood VH. Plant conservation in the Anthropocene: challenges and future prospects. Plant Divers. 2017; 39 :314–330. doi: 10.1016/j.pld.2017.10.004. [ PMC free article ] [ PubMed ] [ CrossRef ] [ Google Scholar ]
- Hoegh-Guldberg O, Poloczanska ES, Skirving W, Dove S. Coral Reef Ecosystems under climate change and ocean acidification. Front. Mar. Sci. 2017; 4 :158. doi: 10.3389/fmars.2017.00158. [ CrossRef ] [ Google Scholar ]
- Hua F, Bruijnzeel LA, Meli P, et al. The biodiversity and ecosystem service contributions and trade-offs of forest restoration approaches. Science. 2022 doi: 10.1126/science.abl4649. [ PubMed ] [ CrossRef ] [ Google Scholar ]
- Hughes TP, Kerry TJ, Baird AH, et al. Global warming transforms coral reef assemblages. Nature. 2018; 556 :492–496. doi: 10.1038/s41586-018-0041-2. [ PubMed ] [ CrossRef ] [ Google Scholar ]
- Immerzeel WW, Lutz AF, Andrade M, et al. Importance and vulnerability of the world’s water towers. Nature. 2020; 577 :364–369. doi: 10.1038/s41586-019-1822-y. [ PubMed ] [ CrossRef ] [ Google Scholar ]
- IPBES: The intergovernmental science-policy platform on biodiversity and ecosystem services. In: Sustainable development goals. IPBES, Bonn (2019)
- IPCC: IPCC special report on carbon dioxide capture and storage (2005). https://www.ipcc.ch›2018/03›srccs_wholereport-1
- Indian State Forest Report: Forest Survey of India (2019). https://www.drishtiias.com
- Jägermeyr J, Müller C, Ruane AC, et al. Climate impacts on global agriculture emerge earlier in new generation of climate and crop models. Nat. Food. 2021 doi: 10.1038/s43016-021-00400-y. [ CrossRef ] [ Google Scholar ]
- Kerr JT, Pinder A, Galpern P, et al. Climate impacts on bumblebees coverage across continents. Science. 2015; 349 :177–180. doi: 10.1126/science.aaa7031. [ PubMed ] [ CrossRef ] [ Google Scholar ]
- King O, Bhattacharya A, Ghuffar S, Tait A, et al. Six decades of glacier mass changes around Mt. Everest are revealed by historical and contemporary Images. One Earth. 2021 doi: 10.1016/j.oneear.2020.10.019. [ CrossRef ] [ Google Scholar ]
- Kudo G. Vulnerability of phenological synchrony between plants and pollinators in an alpine ecosystem. Ecol. Res. 2014; 29 :571–581. doi: 10.1007/s11284-013-1108-z. [ CrossRef ] [ Google Scholar ]
- Kudo G, Ida TY. Early onset of spring increases the mismatch between plants and pollinators. Ecology. 2013; 94 :2311–2320. doi: 10.1890/12-2003.1. [ PubMed ] [ CrossRef ] [ Google Scholar ]
- Lavania UC, Lavania S. Sequestration of atmospheric carbon into subsoil horizons through deep-rooted grasses-vetiver grass model. Curr. Sci. 2009; 97 :618–619. [ Google Scholar ]
- Lee E, Carrivick JL, Quincey DJ, et al. Accelerated mass loss of Himalayan glaciers since the little ice age. Sci. Rep. 2021; 11 :24284. doi: 10.1038/s41598-021-03805-8. [ PMC free article ] [ PubMed ] [ CrossRef ] [ Google Scholar ]
- Leech DI, Crick HQP. Influence of climate change on the abundance, distribution and phenology of woodland bird species in temperate regions. Ibis. 2007; 149 (Suppl. 2):128–145. doi: 10.1111/j.1474-919X.2007.00729.x. [ CrossRef ] [ Google Scholar ]
- Leeming J. Meet the food pioneer whose meat replacements are rocking the gravy boat. Nature. 2021; 590 :176. doi: 10.1038/d41586-021-00264-z. [ PubMed ] [ CrossRef ] [ Google Scholar ]
- Lenzen M, Moran D, Kanemoto K, et al. International trade drives biodiversity threats in developing nations. Nature. 2012; 486 :109–112. doi: 10.1038/nature11145. [ PubMed ] [ CrossRef ] [ Google Scholar ]
- Levin DA. Plant speciation in the age of climate change. Ann. Bot. 2019; 124 :769–775. doi: 10.1093/aob/mcz108. [ PMC free article ] [ PubMed ] [ CrossRef ] [ Google Scholar ]
- Lobell DB, Schlenker W, Costa-Roberts J. Climate trends and global crop production since 1980. Science. 2011; 333 :616–620. doi: 10.1126/science.1204531. [ PubMed ] [ CrossRef ] [ Google Scholar ]
- Lovejoy TE, Hannah L, editors. Biodiversity and climate change: transforming the biosphere. New Haven, London: Yale University Press; 2005. [ Google Scholar ]
- Manisalidis I, Stavropoulou E, Stavropoulos A, Bezirtzoglou E. Environmental and health impacts of air pollution: a review. Front. Public Health. 2020 doi: 10.3389/fpubh.2020.00014. [ PMC free article ] [ PubMed ] [ CrossRef ] [ Google Scholar ]
- Maxwell SL, Butt N, Maron M, et al. Conservation implications of ecological responses to extreme weather and climate events. Divers. Distrib. 2019; 25 :613–625. doi: 10.1111/ddi.12878. [ CrossRef ] [ Google Scholar ]
- McCrystall MR, Stroeve J, Serreze M, et al. New climate models reveal faster and larger increases in Arctic precipitation than previously projected. Nat. Commun. 2021 doi: 10.1038/s41467-021-27031-y. [ PMC free article ] [ PubMed ] [ CrossRef ] [ Google Scholar ]
- Memmott J, Craze PE, Waser NM, Price MV. Global warming and disruption of plant-pollinator interactions. Ecol. Lett. 2007; 10 :710–717. doi: 10.1111/j.1461-0248.2007.01061.x. [ PubMed ] [ CrossRef ] [ Google Scholar ]
- Miller-Rushing A, Hoye TH, Inouye D, Post E. The effects of phenological mismatches on demography. Philos. Trans. R. Soc. B. 2010; 365 :3177–3186. doi: 10.1098/rstb.2010.0148. [ PMC free article ] [ PubMed ] [ CrossRef ] [ Google Scholar ]
- Negrutiu I, Frohlich MW, Hamant O. Flowering plants in the Anthropocene: a political agenda. Trends Plant. Sci. 2020; 25 :349–368. doi: 10.1016/j.tplants.2019.12.008. [ PubMed ] [ CrossRef ] [ Google Scholar ]
- Obura D, Gudka M, Samoilys M, et al. Vulnerability to collapse of coral reef ecosystems in the Western Indian Ocean. Nat. Sustain. 2021; 5 :104–113. doi: 10.1038/s41893-021-00817-0. [ CrossRef ] [ Google Scholar ]
- OXFAM International: 5 natural disasters that beg for climate action. (2021) https://www.oxfam.org/en/5-natural-disasters-beg-climate-action
- Pacheco P, Mo K, Dudley N, et al. Deforestation fronts: drivers and responses in a changing world. Gland: WWF; 2021. [ Google Scholar ]
- Parmesan C, Yohe G. A globally coherent fingerprint of climate change impacts across natural systems. Nature. 2003; 421 :37–42. doi: 10.1038/nature01286. [ PubMed ] [ CrossRef ] [ Google Scholar ]
- Pautasso M. Observed impacts of climate change on terrestrial birds in Europe: an overview. Ital. J. Zool. 2012; 79 :296–314. doi: 10.1080/11250003.2011.627381. [ CrossRef ] [ Google Scholar ]
- Pennisi E. Lyme-carrying ticks live longer—and could spread farther—thanks to warmer winters. Science. 2022 doi: 10.1126/science.acz9985. [ CrossRef ] [ Google Scholar ]
- Pimentel D, Pimentel M. Sustainability of meat-based and plant-based diets and the environment. Am. J. Clin. Nutr. 2003; 78 :660S–663S. doi: 10.1093/ajcn/78.3.660S. [ PubMed ] [ CrossRef ] [ Google Scholar ]
- Pimm SL. Climate disruption and biodiversity. Curr. Biol. 2009; 19 :R595–R601. doi: 10.1016/j.cub.2009.05.055. [ PubMed ] [ CrossRef ] [ Google Scholar ]
- Potapov P, Turubanova S, Hansen MC, et al. Global maps of cropland extent and change show accelerated cropland expansion in the twenty-first century. Nat. Food. 2021 doi: 10.1038/s43016-021-00429-z. [ CrossRef ] [ Google Scholar ]
- Primack RB, Higuchi H, Miller-Rushing AJ. The impact of climate change on cherry trees and other species in Japan. Biol. Conserv. 2009; 142 :1943–1949. doi: 10.1016/j.biocon.2009.03.016. [ CrossRef ] [ Google Scholar ]
- Pruniki M, Cauwenberghs N, Lee J, et al. Air pollution exposure is linked with methylation of immunoregulatory genes, altered immune cell profiles, and increased blood pressure in children. Sci. Rep. 2021; 11 :4067. doi: 10.1038/s41598-021-83577-3. [ PMC free article ] [ PubMed ] [ CrossRef ] [ Google Scholar ]
- Rader R, Reilly J, Bartomeus I, Winfree R. Native bees buffer the negative impact of climate warming on honey bee pollination of watermelon crops. Global Change Biol. 2013; 19 :3103–3110. doi: 10.1111/gcb.12264. [ PubMed ] [ CrossRef ] [ Google Scholar ]
- Raven PH. Biological extinction and climate change. In: Al-Delaimy WK, Ramanathan V, SánchezSorondo M, editors. Health of people, health of planet and our responsibility. Cham: Springer; 2020. pp. 11–20. [ Google Scholar ]
- Razzaq A, Wani SH, Saleem F, et al. Rewilding crops for climate resilience: economic analysis and de novo domestication strategies. J Exptl Bot. 2021; 72 :6123–6139. doi: 10.1093/jxb/erab276. [ PubMed ] [ CrossRef ] [ Google Scholar ]
- Ripple WJ, Wolf C, Newsome TM, et al. World scientists’ warning to humanity: a second notice. Bioscience. 2017; 67 :1026–1028. doi: 10.1093/biosci/bix125. [ CrossRef ] [ Google Scholar ]
- Ripple WJ, Wolf C, Newsome TM, et al. World Scientists’ warning of a climate emergency. Bioscience. 2020; 70 :8–12. doi: 10.1093/biosci/biz152. [ CrossRef ] [ Google Scholar ]
- Salvatteci R, Schneider RR, Field EGD, et al. Smaller fish species in a warm and oxygen-poor Humboldt Current system. Science. 2022; 375 :101–104. doi: 10.1126/science.abj0270. [ PubMed ] [ CrossRef ] [ Google Scholar ]
- Sejiyan V, Bhatta R, Malik PK, et al. Livestock as sources of greenhouse gases and its significance to climate change. In: Moya BL, Pous J, et al., editors. Greenhouse gases. London: IntechOpen; 2016. [ Google Scholar ]
- Shivanna KR. The sixth mass extinction crisis and its impact on biodiversity and human welfare. Resonance. 2020; 25 :93–109. doi: 10.1007/s12045-019-0924-z. [ CrossRef ] [ Google Scholar ]
- Shivanna KR, Sanjappa M. Conservation of endemic and threatened flowering plants: challenges and priorities for India. J. Indian Bot. Soc. 2021; 101 :269–290. [ Google Scholar ]
- Shivanna KR, Tandon R, Koul M. ‘Global pollinator crisis’ and its impact on crop productivity and sustenance of biodiversity. In: Tandon R, Shivanna KR, Koul M, editors. Reproductive ecology of flowering plants: patterns and processes. Singapore: Springer; 2020. pp. 395–413. [ Google Scholar ]
- Sodhi NS, Ehrlich PR, editors. Conservation biology for all. Oxford: Oxford University Press; 2010. [ Google Scholar ]
- Soroye P, Newworld T, Kerr J. Climate change contributes to widespread declines among bumble bees across continents. Science. 2020; 367 :685–688. doi: 10.1126/science.aax8591. [ PubMed ] [ CrossRef ] [ Google Scholar ]
- Stokstad E. World’s largest bean and cassava collection gets a striking new home: “Future Seeds” gene bank will help plant breeders create new varieties of crops and carbon-storing grasses. ScienceInsider. 2022 doi: 10.1126/science.abq1510. [ CrossRef ] [ Google Scholar ]
- Thomas CD. Rapid acceleration of plant speciation during the Anthropocene. Trends Ecol. Evol. 2015; 30 :448–455. doi: 10.1016/j.tree.2015.05.009. [ PubMed ] [ CrossRef ] [ Google Scholar ]
- Velasquez-Tibata J, Salaman P, Catherine H, Graham CH. Effects of climate change on species distribution, community structure, and conservation of birds in protected areas in Colombia. Reg. Environ. Change. 2013; 13 :235–248. doi: 10.1007/s10113-012-032. [ CrossRef ] [ Google Scholar ]
- Villa S, Montagna M, Pierce S. Endemism in recently diverged angiosperms is associated with polyploidy. Plant Ecol. 2022 doi: 10.1007/s11258-022-01223-y9-y. [ CrossRef ] [ Google Scholar ]
- Voosen P. Key Antarctic ice shelf is within years of failure. Science. 2021; 374 :1420–1421. doi: 10.1126/science.acz9833. [ PubMed ] [ CrossRef ] [ Google Scholar ]
- Wagner DL. Insect decline in the Anthropocese. Ann. Rev. Entomol. 2020; 65 :457–480. doi: 10.1146/annurev-ento-011019-025151. [ PubMed ] [ CrossRef ] [ Google Scholar ]
- Wagner, D.L., Grames, E.M., Forister, M.L. et al.: Insect decline in the Anthropocene: Death by a thousand cuts. Proc. Natl. Acad. Sci. USA 118 , e2023989118 (2021). 10.1073/pnas.2023989118 [ PMC free article ] [ PubMed ]
- Warren R, Price J, Graham E, et al. The projected effect on insects, vertebrates and plants of limiting global warming to 1.5°C rather than 2°C. Science. 2018; 360 :791–795. doi: 10.1126/science.aar3646. [ PubMed ] [ CrossRef ] [ Google Scholar ]
- WCRP Global Sea Level Budget Group Global sea-level budget 1993–present. Earth Syst. Sci. Data. 2018; 10 :1551–1590. doi: 10.5194/essd-10-1551-2018. [ CrossRef ] [ Google Scholar ]
- WHO analysis . Monitoring health for the sustainable development goals. Geneva: WHO; 2016. World Health Statistics 2016. [ Google Scholar ]
- Wilson EO. Half-earth: our planet’s fight for life. New York: Liveright/Norton; 2016. [ Google Scholar ]
- Wilson EO, Peter FM, editors. Biodiversity. Washington DC: National Academy Press; 1988. [ PubMed ] [ Google Scholar ]
- Zattara EE, Aizen MA. Worldwide occurrence of records suggest a global decline in bee species richness. One Earth. 2021; 4 :114–123. doi: 10.1016/j.oneear.2020.12.005. [ CrossRef ] [ Google Scholar ]
- Zhao Z, Wang Y, Zang Z, et al. Climate warming has changed phenology and compressed the climatically suitable habitat of Metasequoia Glyptostroboides over the last half century. Global Ecol. Conserv. 2020; 23 :e01140. doi: 10.1016/j.gecco.2020.e01140. [ CrossRef ] [ Google Scholar ]
- Zhu C, Kobayashi K, Loladze I, et al. Carbon dioxide (CO 2 ) levels this century will alter the protein, micronutrients, and vitamin content of rice grains with potential health consequences for the poorest rice-dependent countries. Sci. Adv. 2018; 4 :eaaq012. doi: 10.1126/sciadv.aaq1012. [ PMC free article ] [ PubMed ] [ CrossRef ] [ Google Scholar ]
- Zhu T, Flavio C, De Lima F, De Smet I. The heat is on: how crop growth, development, and yield respond to high temperature. J. Exptl. Bot. 2021; 72 :7359–7373. doi: 10.1093/jxb/erab308. [ PubMed ] [ CrossRef ] [ Google Scholar ]

Dissertations and Theses of Ecology Graduates
Dissertations and theses.
- Doctoral Dissertation Titles
- Master Theses and Reports
Advertisement
Research Progress on endangered plants: a bibliometric analysis
- Review Paper
- Published: 07 March 2022
- Volume 31 , pages 1125–1147, ( 2022 )
Cite this article
- Jie Xu 1 , 3 ,
- Pengnan Xiao 2 ,
- TingTing Li 1 , 3 &
- Zhengxiang Wang ORCID: orcid.org/0000-0001-9514-6712 1 , 3
903 Accesses
10 Citations
Explore all metrics
The rapid extinction of endangered plants (EPs) may lead to the destruction of entire ecosystems, which will seriously threaten the survival and development of humans. Research on endangered plants should be strengthened to scientifically guide the protection of endangered plants. Based on 1635 publications collected from the Web of Science Core Collection ™ (WoS), this paper aims to provide a comprehensive bibliometric run-through and visualization of the subject of EPs. Contingent on influential authors, organizations, top journals and subject category, as well as the most influential papers in the EPs field were discussed in detail. Afterwards, by analyzing the co-occurrence network and evolution path of keywords, to discover the main research topics. In the discussion part, this paper focuses on important issues and research frameworks in the field. The main motive of the paper is to assist research workers interested in the area of EPs to determine the ongoing potential research opportunities and hotspots.
This is a preview of subscription content, log in via an institution to check access.
Access this article
Price includes VAT (Russian Federation)
Instant access to the full article PDF.
Rent this article via DeepDyve
Institutional subscriptions
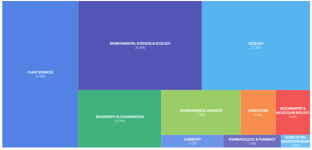
Similar content being viewed by others
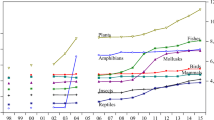
Impact of climate change on biodiversity loss: global evidence
Muzafar Shah Habibullah, Badariah Haji Din, … Hasan Zahid
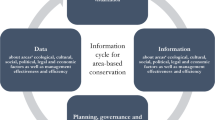
Challenges and opportunities of area-based conservation in reaching biodiversity and sustainability goals
Samuel Hoffmann
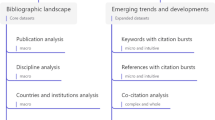
Ecotourism and sustainable development: a scientometric review of global research trends
Lishan Xu, Changlin Ao, … Zhenyu Cai
Data Availability
Not applicable.
Code Availability
Adhikari D, Barik SK, Upadhaya K (2012) Habitat distribution modelling for reintroduction of Ilex khasiana Purk., a critically endangered tree species of northeastern India. Ecol Eng 40:37–43. https://doi.org/10.1016/j.ecoleng.2011.12.004
Article Google Scholar
Bacles CFE, Lowe AJ, Ennos RA (2004) Genetic effects of chronic habitat fragmentation on tree species: the case of Sorbus aucuparia in a deforested Scottish landscape. Mol Ecol 13, 573-584.10.1046/j.1365-294X.2004.02093.x
Barrett S, Kohn JR (1991) Genetic and Evolutionary Consequences of Small Population Size in Plants: Implications for Conservation. Genetics & Conservation of Rare Plants
Caro TM, O. G (1999) On the Use of Surrogate Species in Conservation Biology.Conserv Biol(4),805–814
Caro T, Laurenson M, Karen M (1994) Ecological and genetic factors in conservation: A cautionary tale. Science
Chao S, Wang S, Xia EH, Jiang JJ, Zeng FC, Gao LZ (2016) Full transcription of the chloroplast genome in photosynthetic eukaryotes. Sci Rep-Uk.10.1038/srep30135
Chen L, Gao QK, Chen DM, Xu CJ (2005) The use of RAPD markers for detecting genetic diversity, relationship and molecular identification of Chinese elite tea genetic resources [Camellia sinensis (L.) O. Biodivers Conserv 14:1433–1444
CM C (2006) CiteSpace II: Detecting and visualizing emerging trends and transient patterns in scientific literature. Journal of the American Society for Information Science and Technology (3),359–377. 10.1002/asi.20317
Csergő AM, Salguero Gómez R, Broennimann O, Coutts SR, Guisan A, Angert AL, Welk E, Stott I, Enquist BJ, McGill B, Svenning JC, Violle C, Buckley YM (2017) Less favourable climates constrain demographic strategies in plants. Ecol Lett 20, 969-980.10.1111/ele.12794
Cyranoski D (2008) China: Visions of China. Nature 454, 384-387.10.1038/454384a
Dongyan X (2004) A floristic study of the rare and endangered plants in Baimashan nature reserve. JOURNAL OF HAINAN NORMAL UNIVERSITY 17, 278–281, 302.10.3969/j.issn.1674-4942.2004.03.017
Draper D, Rosselló-Graell A, Garcia C, Gomes CT, Sérgio (2003) C., Application of GIS in plant conservation programmes in Portugal. Biol Conserv 113, 337–349
Tao DU (2017) W. S. Q. X., Analysis of competitiveness of international geographic institutes based on bibliometrics. Acta Geographica Sinica 72, 1702-1716.10.11821/dlxb201709014
Du Xiajin, Hengan Z, Xiaofeng L, Li W (2017) Megasporogenesis and Development of Female Gametophyte of Endangered Plant Diplopanax stachyanthus. Journal of Tropical and Subtropical Botany 25, 605-609.10.11926/jtsb.3696
Eck N, Waltman L (2010) Software survey: VOSviewer, a computer program for bibliometric mapping. Scientometrics 84:523–538
Eggleton LHJB (1998) Biodiversity inventories, indicator taxa and effects of habitat modification in tropical forest. Nature
El-Banhawy A, Nour IH, Acedo C, Elkordy A, Ellmouni Y (2021) Taxonomic Revisiting and Phylogenetic Placement of Two Endangered Plant Species: Silene leucophylla Boiss. and Silene schimperiana Boiss. (Caryophyllaceae) Plants 10:740
Article CAS Google Scholar
Elith J, Graham H, Anderson CP, Dudík R, Ferrier M, Guisan S, Hijmans AJ, Huettmann R, Leathwick FR, Lehmann J, Li A, Lohmann JG, Loiselle LA, Manion B, Moritz G, Nakamura C, Nakazawa M, Overton YMcCM, Townsend Peterson J, Phillips AJ, Richardson S, Scachetti-Pereira K, Schapire RE, Soberón R, Williams J, Wisz SS, Zimmermann ME (2006) N., Novel methods improve prediction of species’ distributions from occurrence data. Ecography 29, 129-151.10.1111/j.2006.0906-7590.04596.x
Engelmann F (2011) Use of biotechnologies for the conservation of plant biodiversity. In Vitro Cellular & Developmental Biology - Plant 47, 5-16.10.1007/s11627-010-9327-2
Fanrong F (2008) Research on Endangered Plant Baiguimu’s Endangered System and Ex-situ Conservation. Fujian Agriculture and Forestry University
Fanrong F, Xiangqing M, Mark P (2008) Research progress in conservation biology of endangered plants in China. CHINA FORESTRY SCIENCE AND TECHNOLOGY, pp 1–5
Farwig N, Braun C, Hning-Gaese B (2008) K., Human disturbance reduces genetic diversity of an endangered tropical tree, Prunus africana (Rosaceae). Conserv Genet 9, 317.10.1007/s10592-007-9343-x
Fenu G, Bacchetta G, Charalambos SC, Fournaraki C, Galdo G, Gotsiou P, Kyratzis A, Piazza C, Vicens M, Pinna MS (2019) An early evaluation of translocation actions for endangered plant species on Mediterranean islands. Plant Diversity 41, 94-104.10.1016/j.pld.2019.03.001
Garfield E, Paris SW, Stock WG (2006) HistCite: A software tool for informetric analysis of citation linkage. NFD Information-Wissenschaft und Praxis 57:391–400
Google Scholar
Gibbs JP (2001) Demography versus habitat fragmentation as determinants of genetic variation in wild populations. Biol Conserv 100, 15-20.10.1016/S0006-3207(00)00203-2
Godefroid S, Piazza C, Rossi G, Buord S, Stevens A, Aguraiuja R, Cowell C, Weekley CW, Vogg G, Iriondo JM, Johnson I, Dixon B, Gordon D, Magnanon S, Valentin B, Bjureke K, Koopman R, Vicens M, Virevaire M, Vanderborght T (2011) How successful are plant species reintroductions? Biol Conserv 144, 672-682.10.1016/j.biocon.2010.10.003
Goodman BD (1990) The demography of chance extinction. Viable Population for Conservation
Guirui Y (2003) Global Change and Carbon Cycle and Carbon Storage in Terrestrial Ecosystem (Fine). Meteorological Press
Guisan A, Broennimann O, Engler R (2006) Using Niche-Based Models to Improve the Sampling of Rare Species. Conserv Biol 20, 501-511.10.1111/j.1523-1739.2006.00354.x
Guisan A, Zimmermann NE (2000) Predictive habitat distribution models in ecology. Ecol Model 135, 147-186.10.1016/S0304-3800(00)00354-9
Guo-hua L, Bo-jie F (2001) Effects of global climate change on forest ecosystems. JOURNAL OF NATURAL RESOURCES, 71-78.10.3321/j.issn:1000-3037.2001.01.013
Honnay O, Jacquemyn H (2007) Susceptibility of Common and Rare Plant Species to the Genetic Consequences of Habitat Fragmentation. Conserv Biol 21, 823-831.10.1111/j.1523-1739.2006.00646.x
Huang J, Chen B, Liu C, Lai J, Zhang J, Ma K (2012) Identifying hotspots of endemic woody seed plant diversity in China. Divers Distrib 18, 673-688.10.1111/j.1472-4642.2011.00845.x
IUCN (2021) IUCN Red List of Threatened Species. IUCN
Jeong HM, Kim HR, Hong S, You YH (2018) Effects of elevated CO2 concentration and increased temperature on leaf quality responses of rare and endangered plants. Journal of Ecology & Environment 42, 1.10.1186/s41610-017-0061-0
Jiakuan HSGY (1998) Variation of flower number and seed setting rate of Liriodendron tulipifera. Acta Botanica Sinica, 27-32.10.3321/j.issn:1672-9072.1998.01.003
Jin’E M (2008) Study on Physiological and Ecological Characteristics of Endangered Plant Sinocalycanthus. Southwest University
Jingyun F (2000) Global Ecology: Climate Change and Ecological Response
Lande MR (1991) Assessing Extinction Threats: Toward a Reevaluation of IUCN Threatened Species Categories. Conserv Biol 5:148–157
Legname G, Nguyen HOB, Baskakov IV, Cohen FE, DeArmond SJ, Prusiner SB (2005) Strain-specified characteristics of mouse synthetic prions. Proceedings of the National Academy of Sciences 102, 2168-2173.10.1073/pnas.0409079102
Liang F, Huang Q, Yanping YU, Liang H, Huang Q, Liu X, Chen Q, Tan X, University YN (2019) Physiological response of endangered semi-mangrove Barringtonia racemosa to salt stress and its correlation analysis. Journal of Central South University of Forestry & Technology 39, 12-18.10.14067/j.cnki.1673-923x.2019.10.003
Li-hua Z, Xiu-zhen C, Hong-liang Z (2006) Endangering Mechanism and Strategy for Conservation of Endangered Plant. Journal of Hunan Institute of Humanities, Science and Technology, pp 43–46. 10.3969/j.issn.1673-0712.2006.06.015
Liu H, Yan MA, Qichao WU, Wang Y, Zang D, University SA (2020) Intraspecific and interspecific competition of the endangered plant Salix taishanensis. Journal of Forest and Environment 40, 178-183.10.13324/j.cnki.jfcf.2020.02.010
Liu N, Zhu W, Sun Z, Yang L, Yuan S, Ren H (2014) Canopy Size Dependent Facilitations from the Native ShrubRhodomyrtus tomentosa to the Early Establishment of Native TreesCastanopsis fissa and Syzygium hancei in Tropical China. Restor Ecol 22, 509-516.10.1111/rec.12094
Liu Z, Shu Q, Wang L, Yu M, Hu Y, Zhang H, Tao Y, Shao Y (2012) Genetic diversity of the endangered and medically important Lycium ruthenicum Murr. revealed by sequence-related amplified polymorphism (SRAP) markers. Biochem Syst Ecol 45, 86-97.10.1016/j.bse.2012.07.017
Liwen W (2016) The value of U.S. national parks. Grand Garden of Science, 3.10.3969/j.issn.1003-1871.2016.22.007
Mark FFMX (2008) Research progress in conservation biology of endangered plants in China. CHINA FORESTRY SCIENCE AND TECHNOLOGY, 1-5.10.3969/j.issn.1000-8101.2008.03.001
Myers N (1988) Myers N. — Threatened biotas: “Hot Spots” in tropical forests. Environmentalist The Environmentalist 8:187–208
Nicotra A, Hoyle G, Steadman K, Good R (2014) Australian alpine seed ecology: plant conservation and adaptation to climate change. ARC linkage project, Symposium on Alpine Seed & Plant Ecology in the Australian Alps
Ning X, Chen LN, Khoon-Meng W, Cui YZ, Yang HQ, Monica S (2016) Seed Set and Natural Regeneration of Dendrocalamus membranaceus Munro after Mass and Sporadic Flowering in Yunnan, China. Plos One 11, e153845.10.1371/journal.pone.0153845
Olde Venterink H, van der Vliet RE, Wassen MJ (2001) Nutrient limitation along a productivity gradient in wet meadows. Plant Soil 234, 171-179.10.1023/A:1017922715903
Orme CDL, Davies RG, Burgess M, Eigenbrod F, Pickup N, Olson VA, Webster AJ, Ding T, Rasmussen PC, Ridgely RS, Stattersfield AJ, Bennett PM, Blackburn TM, Gaston KJ (2005a) Owens, I. P. F., Global hotspots of species richness are not congruent with endemism or threat. Nature 436, 1016-1019.10.1038/nature03850
Orme CDL, Davies RG, Burgess M, Eigenbrod F, Pickup N, Olson VA, Webster AJ, Ding T, Rasmussen PC, Ridgely RS, Stattersfield AJ, Bennett PM, Blackburn TM, Gaston KJ (2005b) Owens, I. P. F., Global hotspots of species richness are not congruent with endemism or threat. Nature 436, 1016-1019.10.1038/nature03850
Petrie M, Bradford D, Hubbard JB, Lauenroth RM, Andrews WK (2017) C., M., Climate change may restrict dryland forest regeneration in the 21st century. Ecology.10.1002/ecy.1791
Quanguang Y, Chai S, Wu R, Zou R, Wei X (2020) Characteristics of Concomitant Community and Population Structure of Endangered Plant Camellia tunghinensis. Guangxi Forestry Science 036, 45–55, 64.10.3969/j.issn.1006-1126.2020.04.003
Rovzar C, Gillespie TW, Kawelo K (2016) Landscape to site variations in species distribution models for endangered plants. Forest Ecol Manag 369, 20-28.10.1016/j.foreco.2016.03.030
Saccheri I, Kuussaari M, Kankare M, Vikman P, Fortelius W, Hanski (1998) I., Inbreeding and extinction in a butterfly metapopulation. Nature 392, 491
Salmerón-Sánchez E, Mendoza-Fernández AJ, Lorite J, Mota JF, Peñas J (2021) Plant conservation in Mediterranean-type ecosystems. Mediterranean Botany 42, e71333.10.5209/mbot.71333
Sarasan V, Cripps R, Ramsay MM, Atherton C, McMichen M, Prendergast G, Rowntree JK (2006) CONSERVATION IN VITRO OF THREATENED PLANTS – PROGRESS IN THE PAST DECADE. In vitro cellular & developmental biology. Plant 42, 206-214.10.1079/IVP2006769
Shao-xian W, Fei W, Cheng-bai L, Zhi-yong Y, Xia C (2008) Application of DNA molecular markers in endangered species conservation.CHINESE JOURNAL OF ECOLOGY,250–256.CNKI:SUN:STXZ.0.2008-02-021
Shengce T, Zhiping Z, Xianen Z (2001) Development of PCR Technology. China Biotechnology 21, 26-29.10.3969/j.issn.1671-8135.2001.04.006
Song G, Haiqun W, Canming Z, Deyuan H (1997) Genetic Diversity and Population Differentiation of Cathaya Argyrophylla in Bamian Mountain. J Integr Plant Biol , 266–271
Stephen B, See-Chung C, Chong SL, Frieda C, Fiona I, Putri WU, Neil W, Wortley AH (2012) Observations on the Morphology, Pollination and Cultivation of Coco de Mer (Lodoicea maldivica (J F Gmel.) Pers., Palmae). Journal of Botany,2012,(2012-3-14) 2012, 1-13.10.1155/2012/687832
Steven S (2013) Plant Reintroduction in a Changing Climate: Promises and Perils. Restor Ecol
Sumin LI, You W, Xiao S, Dongjin HE, Hong W, Liu J (2015) Gap micro-environment of endangered plant Tsuga longibracteata forests in Tianbaoyan National Nature Reserve. Journal of Forest and Environment 35.10.13324/j.cnki.jfcf.2015.04.009
Sun J, Wu Y, He Y (2018) The Geographical Sciences during 1986–2015: From the Classics to the Frontiers
Tingting LCLP (2009) The Niche Characteristics of Endangered Plant Tsuga tchekiangensisin Jiulongshan National Nature Reserve of Zhejiang Province. JOURNAL OF WUHAN BOTANICAL RESEARCH 27, 55-61.10.3969/j.issn.2095-0837.2009.01.009
van Eck NJ, Waltman L (2010) Software survey: VOSviewer, a computer program for bibliometric mapping. Scientometrics 84, 523-538.10.1007/s11192-009-0146-3
Vitousek PM (1994) Beyond Global Warming: Ecology and Global Change. Ecology 75, 1861-1876.10.2307/1941591
Volis S (2016) How to conserve threatened Chinese plant species with extremely small populations? Plant Diversity 38, 45-52.10.1016/j.pld.2016.05.003
Wassen MJ, Venterink HO, Lapshina ED, Tanneberger F (2005) Endangered plants persist under phosphorus limitation. Nature 437, 547-550.10.1038/nature03950
Wassen M, Venterink H, Lapshina E, Tanneberger F (2005) Endangered plants persist under phosphorus limitation. Nature 437, 547-550.10.1038/nature03950
Wei L, Guihua L, Jin Z, Desi (2002) H.,
Studies on the Seed Bank of Freshwater Wetland:A review. ACTA ECOLOGICA SINICA, 395-402.10.3321/j.issn:1000-0933 (2002) 03.016
Whittaker RJ, Araújo MB, Jepson P, Ladle RJ, Watson JEM, Willis KJ (2005) Conservation Biogeography: assessment and prospect. Divers Distrib 11, 3-23.10.1111/j.1366-9516.2005.00143.x
Wilson EO (1988) Biodiversity, National Forum on Biodiversity, Washington D.C. 1986
Xianwei Y, Jie C, Ge, Ying, Guan, Baohua F, Meiying, Qiu Y (2002) A comparison of dormancy and germination of seeds between an endangered species, Changium smyrnioides, and a non-endangered species, Anthriscus sylvestris. Biodivers Sci 10:425–430
Xiao-xue CSYX (2010) Seed germination characteristics of endangered plant Sinia rhodoleuca. Chin J Ecol 29:233–237. doi: https://doi.org/10.7498/aps.62.174102
Xibin ZSBX (2014) Progress in Chinese geography research reflected from Acta Geographica Sinica during 1934–2013:A bibliometrics analysis. Acta Geographica Sinica 69, 1077-1092.10.11821/dlxb201408005
Xin-Hua ZDJ (1999) Progress in studies of genetic diversity and conservation biology of endangered plant species. CHINESE BIODIVERSITY, 31-37.10.3321/j.issn:1005-0094.1999.01.006
Xin-ran W (2015) Application and Prospect of Biotechnology in the Research and Development of Medicinal Plants. Science&Technology Vis 46:2343
Xu L (2017) The application and prospect of biotechnology in the research and development of medicinal plants. REGIONAL GOVENANCE (5).10.3969/j.issn.2096-4595.2017.05.134
YAN ZU YT (1998) ECOLOGICAL ANALYSIS ON SEXUAL REPRODUCTIVE PRODUCE AND ENDANGERED REASON OF RHODIOLA SACHALINENSIS. Plant Res 18:336–340
Yinghong J (2018) Analysis of the ecological characteristics and endangered causes of the extremely small population of Phoebe hainanensis. Central South University of Forestry and Technology
Yong ZYLY (2017) Flower phenology and breeding system of endangered mangrove Lumnitzera littorea (Jack.) Voigt. Chinese Journal of Applied & Environmental Biology 23, 77-81.10.3724/SP.J.1145.2016.03021
Yuanhuo D, Zhou S (2008) The classification of endangered degrees of species and the progress in researches on species- endangering mechanisms. BIOLOGY TEACHING 33, 5-6.10.3969/j.issn.1004-7549.2008.06.002
Ze-xin J, Jun-min L (2007) ISSR analysis on genetic diversity of endangered relic shrub Sinocalycanthus chinensis. Chin J Appl Ecol 18:247–253
Zhihuan H (2020a) The Research Progress of Endangered Causes and Protection Strategy of Rare and Endangered Plants in China. Journal of University of Souht China(Science & Technology) 34, 42-50.10.19431/j.cnki.1673-0062.2020.03.007
Zhihuan H (2020b) The Research Progress of Endangered Causes and Protection Strategy of Rare and Endangered Plants in China. J Univ Souht China(Science Technology) 34:42 -50.10. 19431 /j. cnki
Zhuang XLGT (2011) Pollination biology of Camellia changii. Chinese Journal of Ecology 30, 552-557.10.3724/SP.J.1011.2011.00150
Zimmermann NE, Yoccoz NG, Edwards TC, Meier ES, Thuiller W, Guisan A, Schmatz DR, Pearman PB (2009) Climatic extremes improve predictions of spatial patterns of tree species.P Natl Acad Sci Usa106
Download references
This research was funded by the National Natural Science Foundation of China (42101065), the Central Government Guides Local Science and Technology Development Projects(2019ZYYD050), the Open Foundation of Regional Development and Environmental Response, Key Laboratory of Hubei Province (2021(C)002.)
Author information
Authors and affiliations.
Faculty of Resources and Environmental Science, Hubei University, 430062, Wuhan, China
Jie Xu, TingTing Li & Zhengxiang Wang
The College of Urban & Environmental Sciences, Central China Normal University, 430079, Wuhan, China
Pengnan Xiao
Regional Development and Environmental Response, Key Laboratory of Hubei Province, 430062, Wuhan, China
You can also search for this author in PubMed Google Scholar
Contributions
Conceptualization, ZX.W and J.X.; methodology, J.X and P.X.; software, P.X. and J.X and TT.L.; writing—original draft preparation, P.X. All authors have read and agreed to the published version of the manuscript.”
Corresponding author
Correspondence to Zhengxiang Wang .
Ethics declarations
Statements and declarations.
The authors declare that they have no known competing financial interests or personal relationships that could have appeared to influence the work reported in this paper.
Conflict of interest
The authors declare no conflicts of interest.
Additional information
Communicated by Daniel Sanchez Mata.
Publisher’s Note
Springer Nature remains neutral with regard to jurisdictional claims in published maps and institutional affiliations.
Rights and permissions
Reprints and permissions
About this article
Xu, J., Xiao, P., Li, T. et al. Research Progress on endangered plants: a bibliometric analysis. Biodivers Conserv 31 , 1125–1147 (2022). https://doi.org/10.1007/s10531-022-02392-y
Download citation
Received : 20 June 2021
Revised : 16 January 2022
Accepted : 25 January 2022
Published : 07 March 2022
Issue Date : March 2022
DOI : https://doi.org/10.1007/s10531-022-02392-y
Share this article
Anyone you share the following link with will be able to read this content:
Sorry, a shareable link is not currently available for this article.
Provided by the Springer Nature SharedIt content-sharing initiative
- Research hotspots
- Conservation
- Bibliometrics
- Endangered system
- Find a journal
- Publish with us
- Track your research
- Systematic Map
- Open access
- Published: 11 September 2020
Evidence of the impact of noise pollution on biodiversity: a systematic map
- Romain Sordello 1 ,
- Ophélie Ratel 1 ,
- Frédérique Flamerie De Lachapelle 2 ,
- Clément Leger 3 ,
- Alexis Dambry 1 &
- Sylvie Vanpeene 4
Environmental Evidence volume 9 , Article number: 20 ( 2020 ) Cite this article
44k Accesses
41 Citations
63 Altmetric
Metrics details
A Systematic Map Protocol to this article was published on 12 February 2019
Ecological research now deals increasingly with the effects of noise pollution on biodiversity. Indeed, many studies have shown the impacts of anthropogenic noise and concluded that it is potentially a threat to the persistence of many species. The present work is a systematic map of the evidence of the impacts of all anthropogenic noises (industrial, urban, transportation, etc.) on biodiversity. This report describes the mapping process and the evidence base with summary figures and tables presenting the characteristics of the selected articles.
The method used was published in an a priori protocol. Searches included peer-reviewed and grey literature published in English and French. Two online databases were searched using English terms and search consistency was assessed with a test list. Supplementary searches were also performed (using search engines, a call for literature and searching relevant reviews). Articles were screened through three stages (titles, abstracts, full-texts). No geographical restrictions were applied. The subject population included all wild species (plants and animals excluding humans) and ecosystems. Exposures comprised all types of man-made sounds in terrestrial and aquatic media, including all contexts and sound origins (spontaneous or recorded sounds, in situ or laboratory studies, etc.). All relevant outcomes were considered (space use, reproduction, communication, etc.). Then, for each article selected after full-text screening, metadata were extracted on key variables of interest (species, types of sound, outcomes, etc.).
Review findings
Our main result is a database that includes all retrieved literature on the impacts of anthropogenic noise on species and ecosystems, coded with several markers (sources of noise, species concerned, types of impacts, etc.). Our search produced more than 29,000 articles and 1794 were selected after the three screening stages (1340 studies (i.e. primary research), 379 reviews, 16 meta-analyses). Some articles (n = 19) are written in French and all others are in English. This database is available as an additional file of this report. It provides an overview of the current state of knowledge. It can be used for primary research by identifying knowledge gaps or in view of further analysis, such as systematic reviews. It can also be helpful for scientists and researchers as well as for practitioners, such as managers of transportation infrastructure.
The systematic map reveals that the impacts of anthropogenic noises on species and ecosystems have been researched for many years. In particular, some taxonomic groups (mammals, birds, fishes), types of noise (transportation, industrial, abstract) and outcomes (behavioural, biophysiological, communication) have been studied more than others. Conversely, less knowledge is available on certain species (amphibians, reptiles, invertebrates), noises (recreational, military, urban) and impacts (space use, reproduction, ecosystems). The map does not assess the impacts of anthropogenic noise, but it can be the starting point for more thorough synthesis of evidence. After a critical appraisal, the included reviews and meta-analyses could be exploited, if reliable, to transfer the already synthesized knowledge into operational decisions to reduce noise pollution and protect biodiversity.
For decades, biodiversity has suffered massive losses worldwide. Species are disappearing [ 1 ], populations are collapsing [ 2 ], species’ ranges are changing (both shrinking and expanding) at unprecedented rates [ 3 ] and communities are being displaced by invasive alien species [ 4 ]. All of the above is caused by human activities and scientists regularly alert the international community to our responsibility [ 5 ]. In particular, urban growth is one of the major reasons for biodiversity loss [ 6 , 7 ] in that it destroys natural habitats, fragments the remaining ecosystems [ 8 ] and causes different types of pollution, for example, run-off, waste and artificial light impacting plants and animals [ 9 , 10 ]. Similarly, man-made sounds are omnipresent in cities, stemming from traffic and other activities (industrial, commercial, etc.) [ 11 ] and they can reach uninhabited places [ 12 ]. Anthropogenic noise can also be generated far from cities (e.g. tourism in a national park, military sonar in an ocean, civil aircraft in the sky).
Many studies have shown that such sounds may have considerable impact on animals. However, sound is not a problem in itself. A majority of species hear and emit sounds [ 13 ]. Sounds are often used to communicate between partners or conspecifics, or to detect prey or predators. The problem arises when sounds turn into “noise”, which depends on each species (sensitivity threshold) and on the type of impact generated (e.g. disturbances, avoidance, damage). In this case, we may speak of “noise pollution”. For instance, man-made sounds can mask and inhibit animal sounds and/or animal audition and it has been shown to affect communication [ 14 ], use of space [ 15 ] and reproduction [ 16 ]. This problem affects many biological groups such as birds [ 17 ], amphibians [ 18 ], reptiles [ 19 ], fishes [ 20 ], mammals [ 21 ] and invertebrates [ 22 ]. It spans several types of ecosystems including terrestrial [ 23 ], aquatic [ 24 ] and coastal ecosystems [ 25 ]. Many types of sounds produced by human activities can represent a form of noise pollution for biodiversity, including traffic [ 26 ], ships [ 27 ], aircraft [ 28 ] and industrial activities [ 29 ]. Noise pollution can also act in synergy with other disturbances, for example light pollution [ 30 ].
Despite this rich literature, a preliminary search did not identify any existing systematic maps pertaining to this issue. Some reviews or meta-analyses have been published, but most concern only one biological group, such as Morley et al. [ 31 ] on invertebrates, Patricelli and Blickley [ 32 ] on birds and Popper and Hastings [ 33 ] on fishes. Other syntheses are more general and resemble somewhat a systematic map, but their strategies seem to be incomplete. For instance, Shannon et al. [ 34 ] performed their literature search on only one database (ISI Web of Science within selected subject areas) and did not include grey literature. As another example, we can cite Rocca et al. in 2016, a meta-analysis that limited its population to birds and amphibians and its outcome to vocalization adjustment [ 35 ]. As a consequence, a more comprehensive map, covering all species and ecosystems, all sources of man-made sounds and all outcomes, and implementing a deeper search strategy (e.g. several databases, grey literature included) is needed to provide a complete overview for policy and practice.
This report presents a systematic map of evidence of the impact of noise pollution on biodiversity based on an a priori method published in a peer-reviewed protocol [ 36 ]. It describes the mapping process and the evidence base. It includes aggregate data and tables presenting the characteristics of the selected articles to highlight gaps in the literature concerning the issue. A database was produced in conjunction with this report, containing metadata for each selected article including key variables (species, types of sound, effects, etc.).
Stakeholder engagement
The current systematic map is managed by the UMS Patrimoine Naturel joint research unit funded by the French Biodiversity Agency (OFB), the National Scientific Research Center (CNRS) and the National Museum of Natural History (MNHN), in a partnership with INRAE. Our institutions act on behalf of the French Ecology Ministry and provide technical and scientific expertise to support public policies on biodiversity.
We identified noise pollution as an emergent threat for species and ecosystems that public authorities and practitioners will have to mitigate in the coming years. Indeed, for decades, noise regulations have focused primarily on the disturbances for humans, but we expect that public policies for biodiversity conservation will start to pay more attention to this threat. Already, in 1996, for the first time, the European Commission’s Green Paper on Future Noise Control Policy dealt with noise pollution from the point of view of environmental protection. Quiet areas are also recommended to guarantee the tranquility of fauna in Europe [ 37 ]. Since 2000 in France, an article in the Environmental Code (art. L571-1) has contained the terms “harms the environment” with respect to disturbances due to noise. To achieve these objectives, a knowledge transfer from research to stakeholders is needed for evidence-based decisions. We expect that concern for the impacts of noise pollution on biodiversity will develop along the same lines that it did for light pollution, which is now widely acknowledged by society. Anticipating this progress, we proposed to the French Ecology Ministry that we produce a systematic map of the impacts of noise on biodiversity in view of drafting a report on current knowledge and identifying sectors where research is needed to fill in knowledge gaps.
Objective of the review
The objective of the systematic map is to provide a comprehensive overview of the available knowledge on the impacts of noise pollution on species and ecosystems and to quantify the existing research in terms of the taxonomic groups, sources of noise and impact types studied.
The systematic map covers all species and ecosystems. In that we are currently not able to say exactly when a sound becomes a noise pollution for species (which is precisely why a systematic map and reviews are needed on this topic), this map covers all man-made sounds, regardless of their characteristics (e.g. frequency, speed, intensity), their origin (road traffic, industrial machines, boats, planes, etc.), their environment or media (terrestrial, aquatic, aerial) and their type (infrasound, ultrasound, white noise, etc.), and in most cases here uses the term “noise” or “noise pollution”. It does not include sounds made by other animals (e.g. chorus frogs) or natural events (e.g. thunder, waterfalls). The systematic map deals with all kinds of impacts, from biological to ecological impacts (use of space, reproduction, communication, abundance, etc.). It encompasses in situ studies as well as ex situ studies (aquariums, laboratories, cages, etc.). The components of the systematic map are detailed in Table 1 .
The primary question is: what is the evidence that man-made noise impacts biodiversity?
The secondary question is: which species, types of impacts and types of noise are most studied?
The method used to produce this map was published in an a priori peer-reviewed protocol by Sordello et al. [ 36 ]. Deviations are listed below. The method follows the Collaboration for Environmental Evidence (CEE) Guidelines and Standards for Evidence Synthesis in Environmental Management [ 38 ] unless noted otherwise, and this paper conforms to ROSES reporting standards [ 39 ] (see Additional file 1 ).
Deviation from the a priori protocol published by Sordello et al. [ 36 ]
Method enhancements.
We reinforced the search strategy with:
a search performed on both CORE and BASE, whereas the protocol was limited to a search on only one of these two search engines,
export of the first 1000 hits for each search string run on Google Scholar, whereas the protocol foresaw the export of the first 300 hits,
extraction of the entire bibliography of 37 key reviews selected from the previously provided corpus whereas the protocol did not foresee this option.
Method downgrades
Because of our resource limitations:
we could not extract the design comparator (e.g. CE, BAE, BACE),
we could not split each article included in the map into several entries (i.e. a book with several chapters, a proceeding with multiple abstracts, a study with several species, sources of noise or outcomes). Consequently, we coded the multiple aspects of these articles on one line in the map database.
Search for articles
Searches were performed using exclusively English search terms. The list of search terms is presented below (see “ Search string ”).
Only studies published in English and in French were included in this systematic map, due to limited resources and the languages understood by the map team.
Search string
The following search string was built (see Additional file 2 , section I for more details on this process):
((TI = (noise OR sound$) OR TS = (“masking auditory” OR “man-made noise” OR “anthropogenic noise” OR “man-made sound$” OR “music festival$” OR ((pollution OR transportation OR road$ OR highway$ OR motorway$ OR railway$ OR traffic OR urban OR city OR cities OR construction OR ship$ OR boat$ OR port$ OR aircraft$ OR airplane$ OR airport$ OR industr* OR machinery OR “gas extraction” OR mining OR drilling OR pile-driving OR “communication network$” OR “wind farm$” OR agric* OR farming OR military OR gun$ OR visitor$) AND noise))) AND TS = (ecolog* OR biodiversity OR ecosystem$ OR “natural habitat$” OR species OR vertebrate$ OR mammal$ OR reptile$ OR amphibian$ OR bird$ OR fish* OR invertebrate$ OR arthropod$ OR insect$ OR arachnid$ OR crustacean$ OR centipede$)).
Comprehensiveness of the search
A test list of 65 scientific articles was established (see Additional file 2 , section II) to assess the comprehensiveness of the search string. The test list was composed of the three groups listed below.
Forty relevant scientific articles identified by the map team prior to the review.
Eight key articles identified using three relevant reviews: Brumm, 2010 (two articles) [ 40 ], Cerema, 2007 (three articles) [ 41 ] and Dutilleux and Fontaine, 2015 (three articles) [ 42 ].
Seventeen studies not readily accessible or indexed by the most common academic databases, submitted by subject experts contacted prior to the review (29 subject experts were contacted, 7 responded).
Bibliographic databases
The two databases below were searched (see Additional file 2 , section III for more details on database selection):
“Web of Science Core Collection” on the Web of Science platform (Clarivate) using the access rights of the French National Museum of Natural History, using the search string described above. The search covered SCI-EXPANDED, SSCI, A&HCI, CPCI-S, CPCI-SSH, BKCI-S, BKCI-SSH, ESCI and CCR-EXPANDED (see Additional file 2 , section III for the complete list of citation indexes). A first request was run on 14 December 2018, without any timespan restriction, and returned 7859 citations. Secondly, an update request, restricted to 2019, was performed, using the same search string and citation indexes, on 6 May 2020, to collect the documents published in 2019. 685 citations were exported.
Scopus (Elsevier). The search string described above was adapted to take into account differences in the search syntax (see Additional file 2 , section IV). A first search was run on 14 December 2018, without any timespan restriction, using the access rights of the University of Bordeaux and returned 11,186 citations. Secondly, a new request restricted to 2019 was performed on 6 May 2020, using the same search string, using the access rights of the CNRS, to collect the documents published in 2019. 859 citations were exported.
Web-based search engines
Additional searches were undertaken using the three following search engines (see Additional file 2 , section V for more details):
Google Scholar ( https://scholar.google.com/ ). Due to the limitations of Google Scholar, four search strings were constructed with English terms to translate the search string used for the bibliographic databases described above in a suitable form for Google Scholar. The first searches were performed on 11 June 2019 and the first 1000 citations (as a maximum, when available), sorted by citation frequency, were exported to a .csv file for each of the four search strings. Secondly, an update search was performed on 6 May 2020 with the same four search strings to collect the documents published in 2019; all hits (110) were exported;
BASE ( https://www.base-search.net ). Searches were performed on 12 April 2019. Given certain limitations of this search engine (maximum number of string characters), the search string built for the bibliographic databases described above was split into two search strings. Searches were performed on the titles of the articles, with no restriction to open access articles, on all types of documents and without any timespan restriction. The first 300 citations, sorted by relevance, were exported for each of the two search strings to a .csv file;
CORE ( https://core.ac.uk/ ). Searches were performed on 12 February 2019. The search engine allowed the use of the original search string used for the bibliographic databases. Searches were performed on the title of the articles and without any timespan restriction. The first 327 articles were manually downloaded, excepting the duplicates and the dead links.
Specialist websites
The following websites were manually searched for relevant articles, including grey literature:
Achieve QUieter Oceans by shipping noise footprint reduction website: http://www.aquo.eu/ .
Association for biodiversity conservation: http://www.objectifs-biodiversites.com .
Document portal of the French Ecology Ministry: http://www.portail.documentation.developpement-durable.gouv.fr/ .
Document database of the French General commission for sustainable development: http://temis.documentation.developpement-durable.gouv.fr/ .
European Commission websites: http://ec.europa.eu/ and http://publications.jrc.ec.europa.eu/ .
European parliament website: http://www.europarl.europa.eu/ .
French forum against noise: https://assises.bruit.fr/ .
Information and Documentation Center on Noise: http://www.bruit.fr .
We collected nine articles from these specialist websites that we included in the mapping process.
Supplementary searches
A call for literature was conducted via different channels from January 2019 to April 2019 to find supplementary literature, in particular non peer-reviewed articles, published in French or in English.
Specialized organizations were contacted via their networks, their web forums or their mailing lists:
the “IENE—Infra Eco Network Europe” ( http://www.iene.info/ ),
the French program on transportation infrastructure ITTECOP “Infrastructures de Transports Terrestres, ECOsystèmes et Paysages” ( http://www.ittecop.fr/ ),
the French national council for the protection of nature “Conseil national de protection de la nature (CNPN)”,
the Green and blue infrastructure policy, a French public policy ( http://www.trameverteetbleue.fr ),
the “Société Française d’Ecologie” ( https://www.sfecologie.org/ ),
the French national mailing list EvolFrance managed by INRAE on biological evolution and biodiversity ( https://www6.inra.fr/reid_eng/News/Evolfrance ).
The following social media were also used to alert the research community to the systematic map and to request non peer-reviewed articles: ResearchGate ( http://www.researchgate.net ), Twitter ( http://www.twitter.com ), LinkedIn ( http://www.linkedin.com ).
A total of 83 articles were sent to us in response to the call for literature.
Bibliographies from relevant reviews
After having collected the literature from the different sources described above, we selected 37 relevant reviews from our corpus. Then, we extracted all their bibliographic references, resulting in 4025 citations (see the list of the 37 reviews and their corresponding number of extracted citations in Additional File 3 ). Among these citations we excluded all duplicates (intra-duplicates and duplicates between these bibliographies and our previous literature collection). We screened the titles of the remaining citations, we retrieved the pdf file of the selected titles and then we screened their full-texts.
Testing the comprehensiveness of the search results
Among the 65 articles included in the test list, the number of articles retrieved from the main sources are (see Additional file 4 for more details on the comprehensiveness values): WOS CC 55, Scopus 56, Google Scholar 41, CORE 5, BASE 3, Relevant reviews 43.
The low comprehensiveness levels reached with CORE and BASE can be explained by the fact that these two search engines index mostly grey literature (they were included in the search strategy for this reason) such as reports, theses or books, whereas this type of literature is absent from the test list that mainly contains journal articles.
The overall comprehensiveness of the map search strategy is 95% (62 articles out of the 65 articles in the test list were retrieved by the different bibliographic sources, see in Additional file 4 the 3 unretrieved articles).
Manually added articles
Finally, some articles were added manually to the corpus:
the 3 articles included in the test list that were not retrieved by the search strategy,
36 relevant articles identified by the team that were found in other publications, but not retrieved by the search strategy. For example, these articles were detected in proceedings or books from which other articles had already been added to the map and that we discovered during the screening process or the full-text collection.
Duplicate removal
Duplicate removal was carried out throughout the mapping process using Excel (duplicate conditional formatting and visual identification line by line). Duplicates were removed from each corpus (e.g. intra Scopus duplicates) and between bibliographic sources (e.g. duplicates between Scopus and Google Scholar). The selected citation was systematically the one from Web of Science Core Collection because the metadata linked to the citations extracted from this database are more complete compared to the Scopus database and supplementary literature sources (BASE, CORE, Google Scholar, call for literature).
Article screening and study-eligibility criteria
Screening process.
Using the predefined inclusion/exclusion criteria detailed below, all articles were screened using Excel, first on titles, then on abstracts and finally on the full-texts.
When there was any doubt regarding the presence of a relevant inclusion criterion or if there was insufficient information to make an informed decision, articles were retained for assessment at a later stage. In particular, articles retained after title screening, but that did not have an abstract were immediately transferred to full-text screening. Given that titles and abstracts in grey literature do not conform to scientific standards, assessment of grey literature was performed during the full-text screening phase. Care was taken to ensure that reviewers never screened their own articles.
The three screening stages were conducted by three reviewers (RS, SV, AD). To assess the consistency of the inclusion/exclusion decisions, a Randolph’s Kappa coefficient was computed before screening the full search results. To that end, a set of articles was randomly selected (respectively composed of 200 articles for title screening, 20 articles for abstract screening and 15 articles for full-text screening) and screened by each reviewer independently. The process was repeated until reaching a Kappa coefficient value higher than 0.6. But even after reaching the necessary Kappa value, all disagreements were discussed and resolved before beginning the screening process.
During calibration of the map protocol, a scoping stage was conducted in the “Web of Science Core Collection” and the three stages of the screening process were tested by one reviewer (RS) in order to refine the eligibility criteria. For these articles, a second reviewer (SV) examined all the rejected articles. Disagreements were discussed and, in some cases, articles were re-included. At the title screening stage, 4692 titles rejected by RS were checked by SV and 156 (3%) were re-included. At the abstract screening stage, 180 abstracts rejected by RS were checked by SV and none were re-included. At the full-text screening stage, 95 full-texts rejected by RS were checked by SV and none were re-included.
Eligibility criteria
Article eligibility was based on the list of criteria detailed in Table 2 , with no deviation from the a priori protocol.
The language was considered as an eligibility criteria only at the full-text screening stage. This means that if an article had an abstract written in another language than French or English, it was not excluded for this reason and it was transferred to the full-text screening stage.
During the three screening stages, rejected articles were systematically classified into four categories (see Table 3 for examples). When an article topic obviously lay outside the scope of this map, it was marked “D” (for Diverse); otherwise it was marked P for irrelevant Population, E for irrelevant Exposure or O for irrelevant Outcome.
Study-validity assessment
No study validity assessment was performed because the intention of the map was not to examine the robustness of the study designs. Critical appraisals of study validity are usually conducted in the case of systematic reviews, not for systematic maps. Footnote 1
Data-coding strategy
All the articles passing the three screening stages were included in the mapping database, apart from those published in 2019 or 2020. This is because some literature searches did not cover 2019 and others covered only a part of it. Consequently, we decided not to include articles published in 2019 (or in 2020) to maintain consistency in the map statistics. Accepted full-texts published in 2019 or 2020 were not coded and were grouped in an additional file for a possible later update of the map.
Each article included in the map was coded based on the full-text using keywords and expanded comment fields describing various aspects. The key variables are:
Article description:
Article source (WOS research, Scopus research, Google Scholar research, etc.);
Basic bibliographic information (authors, title, article date, journal, DOI, etc.);
Language (English/French);
Article type (journal article, book, thesis, conference object, etc.);
Article content (four possibilities: study, review, meta-analysis, other). A study consists of an experiment or an observation, it can be field based (in situ or ex situ) or model based. A review is a collection of studies, based or not on a standardized method. A meta-analysis is a statistical analysis based on several previously published studies or data;
Article characteristics:
Type of population (taxonomic groups). First, we classified the articles according to four taxa: prokaryotes, vertebrates, invertebrates and plants. Then, for vertebrates and invertebrates, we classified the articles as concerning respectively amphibians/birds/fishes/mammals/reptiles/others or arachnids/crustaceans/insects/mollusks/others. This classification is based on different prior evidence syntheses on noise pollution [ 34 , 53 , 54 ], including more details concerning invertebrates. In addition, it is usual in biodiversity documentation and facilitates understanding by stakeholders;
Type of exposure (sources of noise, see Fig. 1 for more details);
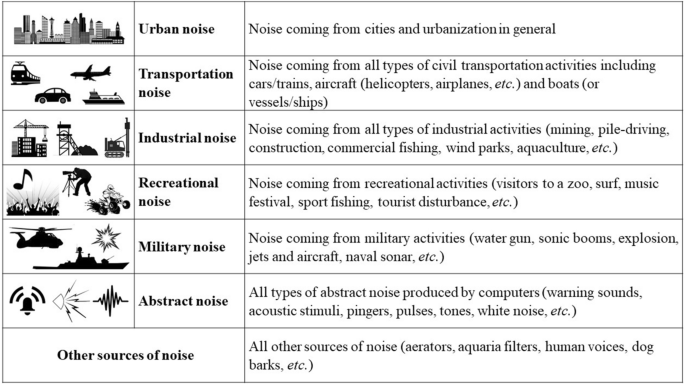
Categories to code the sources of noise (exposure)
Type of outcomes (types of impacts, see Fig. 2 for more details).
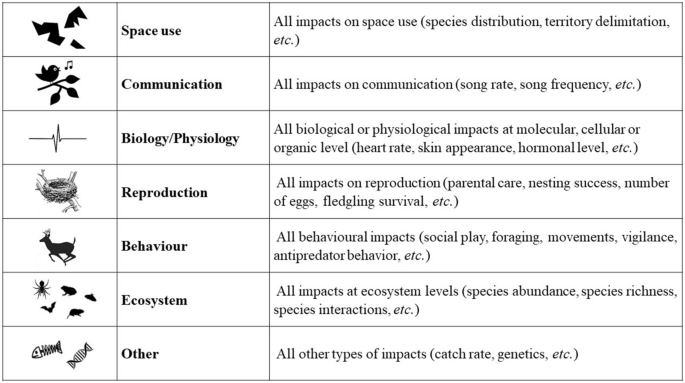
Categories to code the impacts of noise (outcomes)
Here again, to categorize the exposure (sources of noise) and the outcomes (types of impacts), we used previously published evidence syntheses on noise pollution and biodiversity, in particular the review by Shannon et al. (2016) (see in this publication Table 2 , page 988 on the sources of noise and Table 3 , page 989 on the impacts of noise) [ 34 ].
For studies only:
Country where the study was conducted;
Type of habitat (terrestrial or aquatic);
Study context: in situ (field)/ex situ (laboratory, aquariums, etc.);
Experimental (causal)/observational (correlative) study;
Origin of noise (artificial, real, recorded).
These metadata were coded according to an a priori codebook (see Additional file 6 in Sordello et al. [ 36 ]) that was marginally adjusted. The final version of this codebook is included as a sheet in the provided database file (see below the corresponding Additional file 9 ).
As far as possible, controlled vocabularies were used to code the variables (e.g. article type, dates, country, etc.), using thesauri or ISO standards (e.g. ISO 639-1 for the language variable and the ISO 3166-1 alpha 3 code for the country).
Coding was performed by three coders (OR, AD and RS). Because of time and resource limitations in our project, we could not undertake double coding and not all the articles could be coded by a single coder. Coding was carried out by three persons who successively coded a part of the articles. RS began, AD continued and OR finished. One coder coded all variables for the articles included in his/her group of articles (i.e. an article was not coded by several coders). There was no overlap in article coding. To understand the coding rules, explanation was given by RS to AD and OR before they started to code their group of articles. Also, to better understand the coding rules, AD could use the articles previously coded by RS and OR could use the articles previously coded by RS and AD. The three coding steps were monitored by RS who discussed with the two other coders in case of doubt. Finally, when the three groups of articles had been coded, RS reviewed the entire database to identify any errors and homogenize the terminology.
Data-mapping method
By cross-tabulating key meta-data variables (e.g. population and outcomes), summary figures and tables of the article characteristics were produced for this map report to identify knowledge gaps (un- or under-represented subtopics that warrant further primary research) and knowledge clusters (well-represented subtopics that are amenable to full synthesis by a systematic review). Based on these results, recommendations were made on priorities for policy makers, practitioners and research.
Literature searches and screening stages
During the screening process, reviewers did not screen articles that they had authored themselves, except the protocol of this systematic map and it was excluded during the title-screening stage.
The ROSES flow diagram below (Fig. 3 ) provides an overview of the screening process and shows the volumes of articles at the different stages. Detailed screening results are explained in Additional file 5 and illustrated with a full flow diagram in Additional file 6 . The list of all collated and screened articles is provided as an Excel sheet attached to this map report (Additional file 7 ). It contains information on the three screening stages (names of screeners, date of screening, inclusion/exclusion decisions, reason for exclusion, etc.). This file was drafted according to a codebook that describes each variable and the available values and that is included as a sheet in the provided file. In a separate sheet, it also contains the list of excluded full-texts and the reason for exclusion.
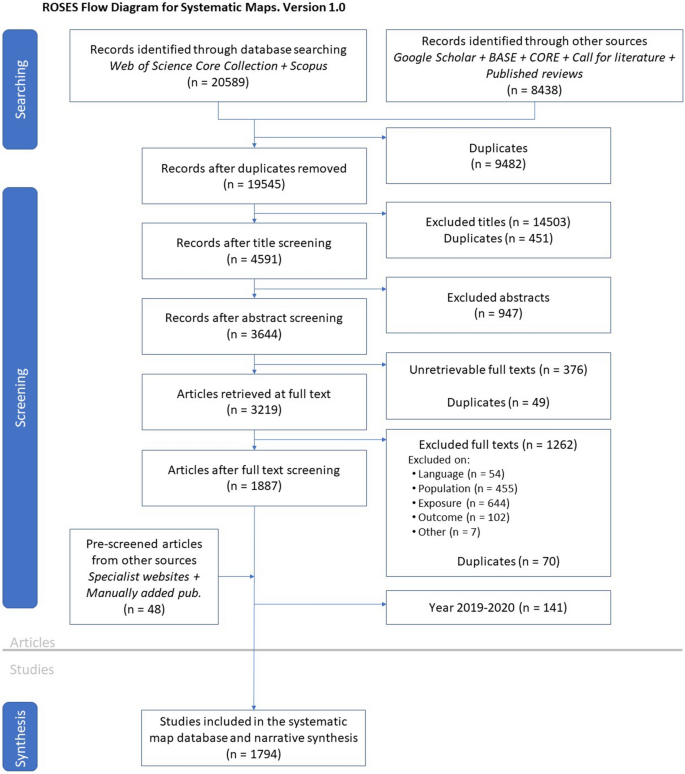
ROSES flow diagram of the systematic map process from the searching stage to the map database. Details are given in the Additional files 5 and 6
Among the 29,027 articles initially collected, 9482 were deleted because they were duplicates, 14,503 were excluded on titles, 947 on abstracts and 1262 on full-texts. A total of 1887 articles were definitively selected after the three screening stages. Among them, 1746 were included in the map to be coded (with 48 more articles manually added or coming from specialist websites) and 141 were grouped in a separate additional file because they were published in 2019–2020 (Additional file 8 ). The systematic-map database contains 1794 relevant articles on the impacts of anthropogenic noises on species and ecosystems (Additional file 9 ), of which 19 are written in French and 1775 in English.
General bibliometrics on the database
Article sources.
The systematic-map database is composed of 1794 articles that come (see Table 4 ):
mainly from bibliographic databases: 65% (48% from WOS CC and 17% from Scopus);
from the bibliography of relevant reviews in a significant proportion: 19%;
from web-based search engines: 12% (in particular 8% from Google Scholar).
Articles coming from the call for literature or the specialist websites and manually added articles represent less than 5% of the map.
Regarding the efficiency of the searches, the call for literature, CORE search engine and Web of Science CC database stand out as the most relevant sources of bibliography for this map (Table 4 ). For instance, 27% of the literature received from the call was included in the map as was 15% from CORE, however these two sources represent a very small part of the final map (1% and 3%, respectively). On the contrary, articles collected from Scopus represent 17% of the final map whereas only 3% of the total number of articles collected from this database were actually relevant. Concerning the key reviews from which citations were extracted, some of these reviews proved to be very useful for the map. For instance, 30% of the bibliography (47 articles) from Gomez et al. [ 55 ] were included in the map (see Additional file 3 for the percentage of extracted/included citations for each key review).
Article types and contents
Figure 4 a shows the distribution of article types. The systematic-map database is mainly composed of journal articles (1333, which represent more than 74%). The second highest proportions of article types in the map are book chapters and reports that each represent 8% of the map.
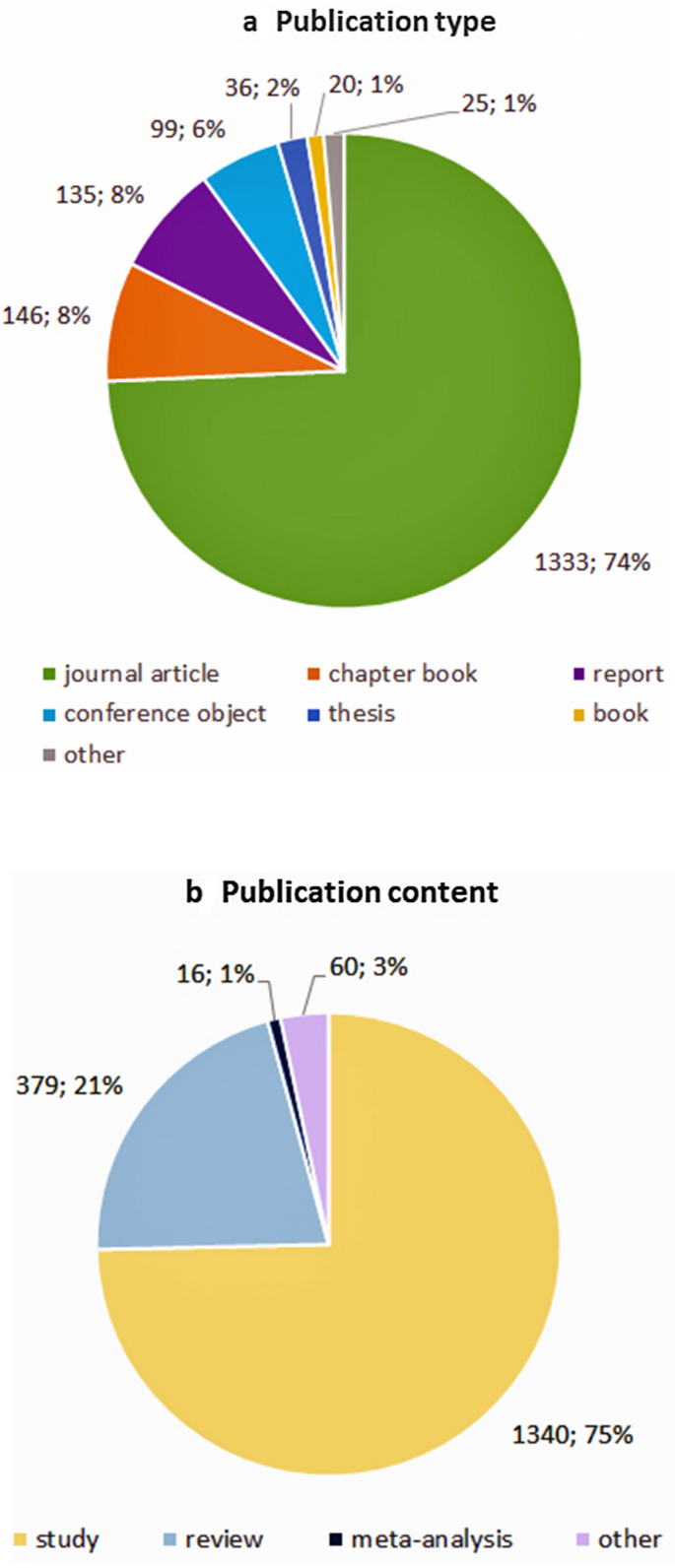
Types ( a ) and contents ( b ) of articles included in the systematic-map database
Figure 4 b shows the distribution of article contents. The systematic-map database is mainly composed of studies (1340, which represent more than 75% of the map), then, reviews (379, 21%) and meta-analyses (16, 1% with one article that is a mixed review/meta-analysis).
Not surprisingly, the majority of studies (1096/1340, 82%) and meta-analyses (13/16, 81%) were published as journal articles. Reviews are more spread over the different types of bibliographic sources even if they are also mainly published as journal articles (186/379, 49%).
Chronological distribution
The systematic-map database contains articles from 1932 to 2018 included. Figure 5 shows that production truely started around 1970 and then strongly increased starting around 2000 (Fig. 5 ).
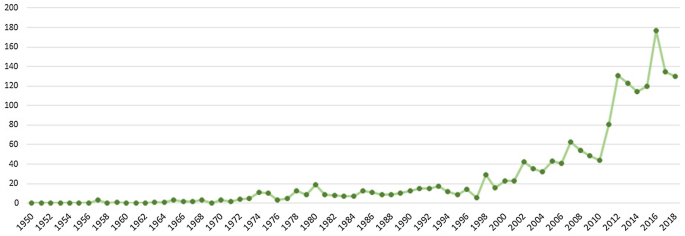
Chronologic number of articles since 1950
Map characteristics on the population, exposure and outcomes
Taxonomic groups.
The systematic map contains articles almost exclusively on vertebrates (1641/1794, 91%). Invertebrates represent 9% of the map and plants and prokaryotes together form less than 1% (however, it should be noted here that our search string did not include “plant” nor “prokaryote” which may partly explain these results).
Mammals, birds and fishes are the three most studied taxonomic groups in the map (see Fig. 6 ), with respectively 778/1794 (43%), 524/1794 (29%) and 437/1794 documents (24%) (the sum of mammals, birds and fishes exceeds the number of vertebrates because one article counted as “vertebrates” can include several vertebrate sub-groups).
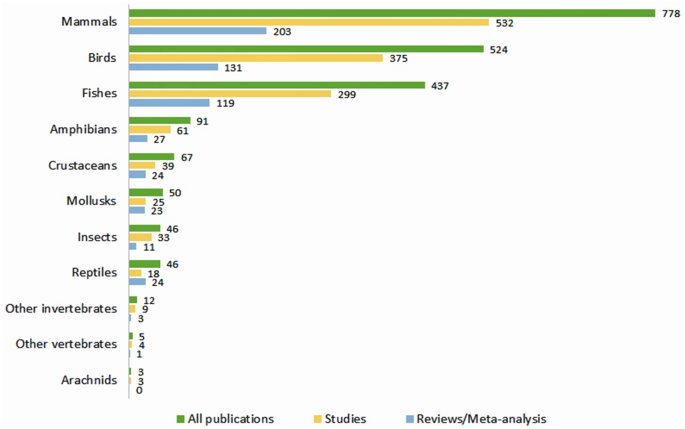
Number of articles for each type of taxonomic group (population), with details for studies and reviews/meta-analyses
These observed patterns regarding the population for the whole map are the same for studies and for reviews/meta-analyses. Mammals, birds and fishes are also the three taxonomic groups most considered in the studies (respectively 40%, 28% and 22%) and in the reviews/meta-analyses (respectively 52%, 33%, 30%).
Among invertebrates, crustaceans represent the most examined group (4% of the map, 3% of the studies, 6% of the reviews/meta-analyses) followed closely by mollusks.
Sources of noise
For 69 articles (4%), we could not precisely code the source of noise in any exposure class. Indeed, these articles use imprecise expressions such as “anthropogenic noise”. Among the others, 619 articles (35% of the map, see Fig. 7 ) deal with transportation noise, followed by industrial noise (27%) and abstract noises (25%). Few articles deal with recreational noise (5% of the map).
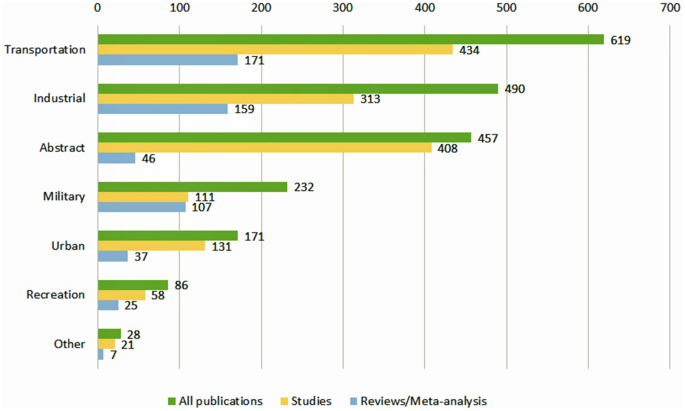
Number of articles for each source of noise (exposure) with details for studies and reviews/meta-analyses
Focusing on the 1340 studies, transportation noise (32%), abstract noise (30%) and industrial noise (23%) are also the three sources of noise most considered, but the ranking was different from that found for all articles. Regarding the reviews/meta-analyses, transportation (43%) and industry (40%) are the two first sources of noise most considered and military noise (27%) comes in as the third source instead of abstract noises.
Types of impacts
The articles included in the map mainly deal with behavioural impacts of noise (985/1794, 55% of the map, see Fig. 8 ). Biophysiology is also frequently considered in the articles (704/1794, 39%) and then communication (424/1794, 24%). For 19 articles (1% of the map) we could not code the outcome because it was not detailed by the authors.
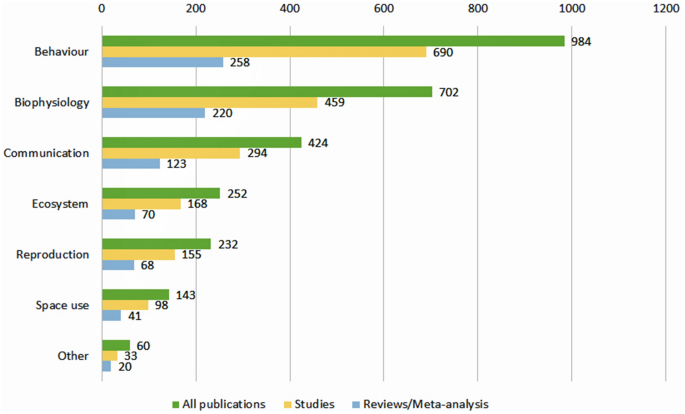
Number of articles for each type of impact (outcomes), with details for studies and reviews/meta-analyses
With a focus on the 1340 studies, impacts of noise on behaviour (51%), on biophysiology (34%) and on communication (22%) are the most considered, similar to the situation for reviews/meta-analyses (respectively 66%, 56% and 31%). On the contrary, space use is the least studied outcome.
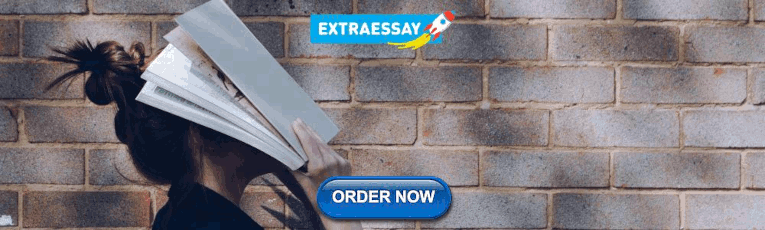
Knowledge gaps and knowledge clusters
We combined the results (number of studies) between two of the three characteristics (population, exposure and outcome), resulting in Figs. 9 , 10 and 11 .
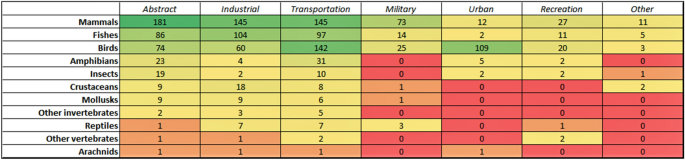
Taxonomic groups (P) and sources of noise (E) in studies
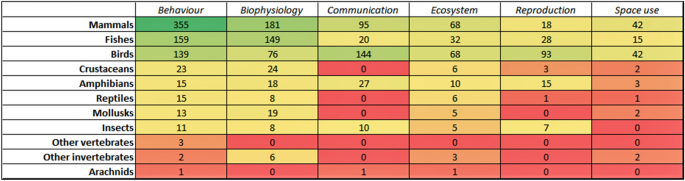
Taxonomic groups (P) and types of impacts (O) in studies

Sources of noise (E) and types of impacts (O) in studies
For each of the three combinations of data, we extracted the top four results (those with the highest number of studies), resulting in 12 knowledge clusters presented in Table 5 . This analysis confirms the knowledge clusters previously noted in the results on population (in Fig. 6 , namely mammals, birds, fishes), exposure (in Fig. 7 , transportation, industrial, abstract noises) and outcomes (in Fig. 8 , behaviour, biophysiology and communication).
Concerning knowledge gaps, the analysis between population, exposure and outcomes reveals that many combinations have never been studied and it is difficult to identify any knowledge gaps in particular. We can refer to separate results on population, exposure and outcomes that show that few studies were conducted on amphibians (61), reptiles (18), all invertebrates (in particular arachnids: 3) and plants (8) in terms of population (see Fig. 6 ); recreational (57), military (106) and urban noises (131) in terms of exposure (see Fig. 7 ); space use (94), reproduction (149) and ecosystems (167) in terms of outcomes (see Fig. 8 ).
Study characteristics
Study location.
Almost one third of all studies (441/1340, 33%) were carried out in the USA (Fig. 12 ). A substantial proportion of the studies were also conducted in Canada (121/1340, 9%), Great Britain (84/1340, 6%), the Netherlands (70/1340, 5%) and even Australia (698/1340, 5%). The country is unknown in 135 studies (10%).
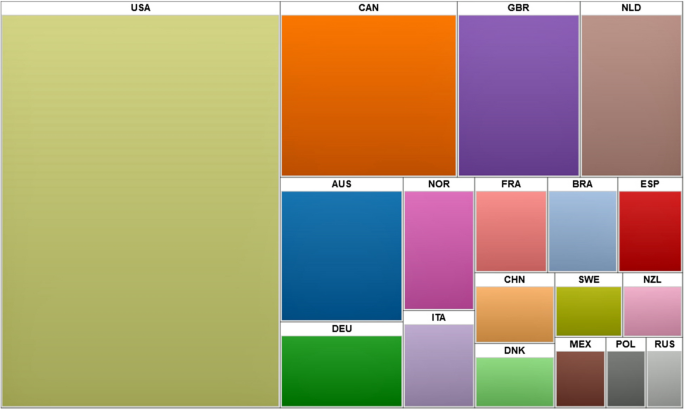
Tree-map representation of the countries where at least 10 studies were included in the map. Values: USA: 441; CAN (Canada): 121; GBR (Great Britain): 84; NLD (Netherlands): 70; AUS (Australia): 69; DEU (Germany): 41; NOR (Norway): 37; FRA (France): 27; ITA (Italia): 27; BRA (Brazil): 26; ESP (Spain): 24; CHN (China): 22; DNK (Denmark): 20; SWE (Sweden): 17; NZL (New-Zealand): 15; MEX (Mexico): 14; POL (Poland): 11; RUS (Russia): 10
Noise source and media
Studies mainly deal with real noise (632/1340, 47%). Around a third of the studies (378/1340, 28%) are based on artificial noise and 16% of the studies (221/1340) use real recorded noise (Fig. 13 a top). The distribution between terrestrial or aquatic media through which noise is broadcast is virtually equivalent (see Fig. 13 b bottom, respectively 47% and 51%).
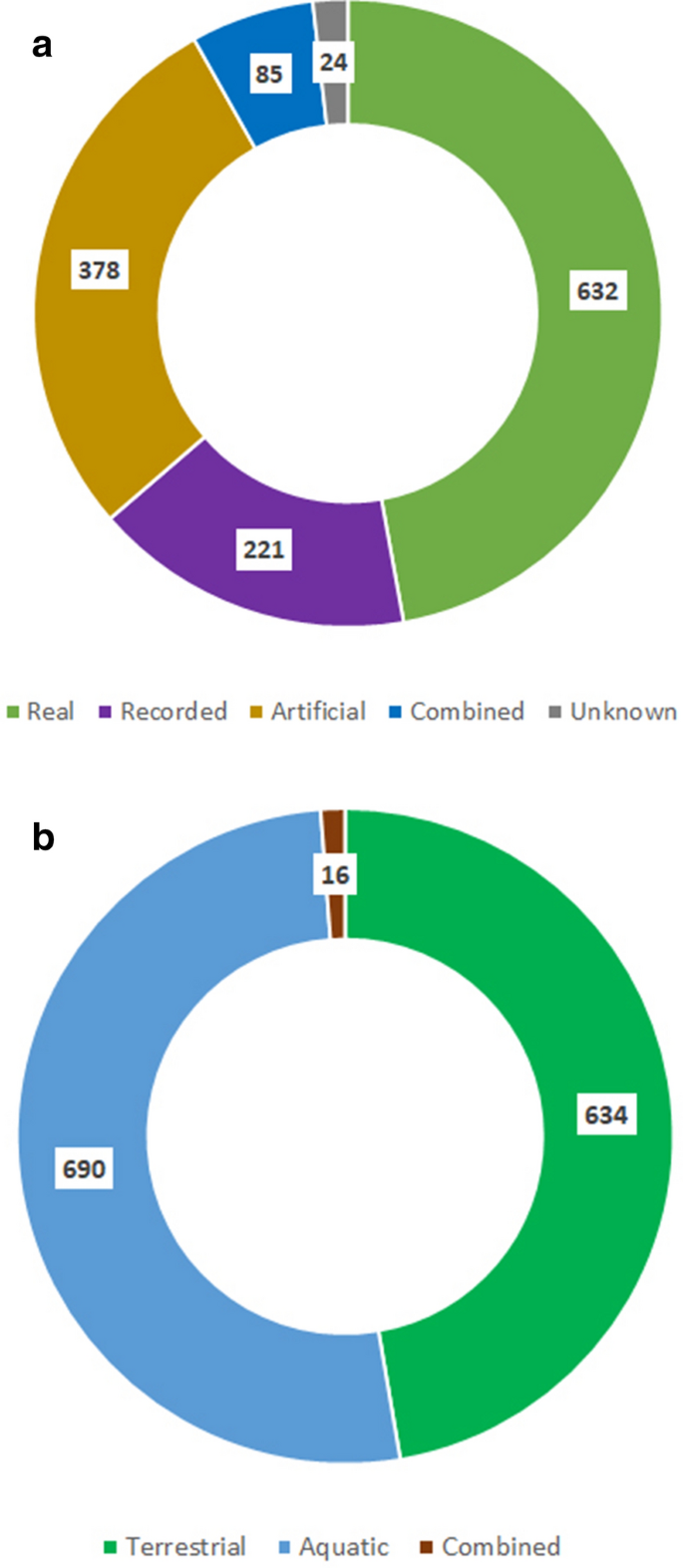
Number of studies included in the map in terms of the noise generated (a; top) and noise media (b; bottom)
Study context and design
Figure 14 shows that 95% of studies (1274/1340) are field based whereas only 3% (40/1340) are model based and less than 1% (9/1340) are combined (field and model based studies). Among the 1283 studies that are totally or partially field based, 56% (720) are in situ whereas 42% (537) are ex situ (zoos, aquarium, cages, etc.) and 2% (26) are combined (Fig. 14 left). Also, a majority are experimental (856/1283, 67%), 32% (411/1283) are observational and less than 1% (12/1283) are combined (experimental and observational) (Fig. 14 right).
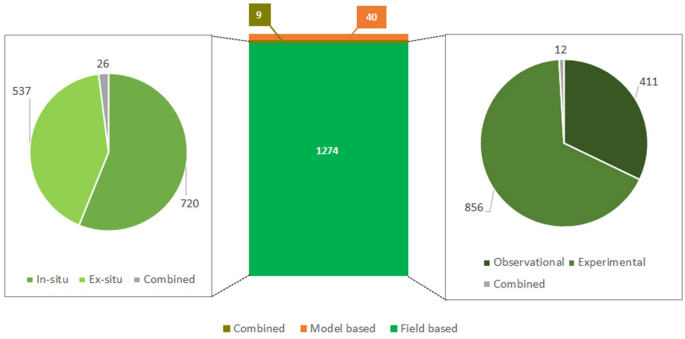
Number of studies included in the map in terms of the context and design protocol
Reviews and meta-analyses
The high number of reviews included in the systematic map (379) can be explained by our methodology. Indeed, some articles were retrieved by our search strategy because they contain only one chapter or one paragraph that reviews the bibliography on impacts of anthropogenic noise on biodiversity. As a consequence, they were included in the map during the screening process even if the document as a whole does not deal with our map’s main issues. Nevertheless, the map does include many reviews that fully address the impacts of noise pollution on species and ecosystems. This means that, contrary to what was assumed beforehand, a huge amount of synthesis work has in fact already been invested in this topic. However, our results confirm that, for the moment, no prior systematic map—as broad and comprehensive as the present one—has been published yet, even if after the date of our literature search, a systematic-map protocol has been published on the impact of noise, focusing on acoustic communication in animals [ 56 ].
Some of the collected reviews are general syntheses and provide an overview of the impacts of anthropogenic noise on species (i.e. Kight and Swaddle [ 57 ]; Dufour [ 58 ]). However, most of reviews are focused on one or more population(s), exposure(s) and outcomes(s) or even a combination of these three parameters. For instance:
concerning taxonomic groups (population): some reviews deal with specific taxa—such as fishes [ 59 ], marine mammals [ 60 ] or crustaceans [ 61 ]—or with wider groups—such as invertebrates [ 31 ] or even terrestrial organisms [ 62 ];
concerning types of noise (exposure): Pepper et al. [ 63 ] address aircraft noise, Patricelli and Blickley [ 32 ] urban noise and Larkin [ 64 ] military noise;
concerning types of impacts (outcomes): De Soto et al. [ 65 ] (which is a proceeding) focus on physiological effects, Brumm and Slabbekoorn [ 66 ] target communication and Tidau and Briffa [ 67 ] (which is also a proceeding) deal with behavioural impacts.
Five reviews are presented as “systematic reviews” by their authors. One of them is Shannon et al. [ 34 ], which is indeed a wide synthesis of the effects of noise on wildlife. Another is dedicated to behavioural responses of wild marine mammals and includes a meta-analysis (quantitative synthesis) [ 55 ]. Two other systematic reviews include noise effects in a wider investigation of the impacts of some human activities, respectively seismic surveys [ 68 ] and wind energy [ 69 ]. The fifth is more specific and deals with the impact of prenatal music and noise exposure on post-natal auditory cortex development for several animals such as chickens, rats, mice, monkeys, cats and pigs [ 70 ]. Two other reviews—Radford [ 54 ] and Williams et al. [ 71 ]—could be qualified as “systematic” because their method is standardized (e.g. search string, screening process), but their authors have not done so.
Among the meta-analyses included in the map, we can cite in particular Cox et al. [ 72 , 73 ] on fishes, Roca et al. [ 35 ] on birds and anurans and Gomez et al. [ 55 ] on marine mammals. Birds are particularly considered since two more meta-analyses deal with this taxonomic group [ 74 , 75 ]. We can also note Cardoso et al. [ 76 ] on the impact of urban noise on several species.
Finally, regarding books, five of them are particularly relevant to the map topic, chronologically:
“Effects of Noise on Wildlife” [ 77 ];
“Marine Mammals and Noise” [ 78 ];
“Animal Communication and Noise” [ 79 ];
“The Effects of Noise on Aquatic Life” (Popper and Hawkins), published in two volumes 2012 and 2016 [ 80 , 81 ];
“Effects of Anthropogenic Noise on Animals” [ 82 ] which is the newest book on noise pollution and wildlife with syntheses for taxonomic groups such as fishes [ 83 ], reptiles and amphibians [ 84 ], birds [ 85 ] and marine mammals [ 86 ].
Some other books can be very general in discussing noise pollution, for instance “Railway ecology” [ 87 ]. Lastly, some other books can contain entire chapters specifically on noise pollution, e.g. “Avian Urban Ecology: Behavioural and Physiological Adaptations” [ 88 , 89 ] or “The Handbook of Road Ecology” [ 90 , 91 ]. We can also cite the “Ornithological Monographs” N°74 which is dedicated to noise pollution and contains one review [ 92 ] and several studies that are all included in the map [ 93 , 94 ].
Recently, some relevant syntheses were published in 2019 (not included in the map; see Additional file 8 ). A meta-analysis was performed on the effects of anthropogenic noise on animals [ 53 ] and a systematic review was published on intraspecific variation in animal responses to anthropogenic noise [ 95 ]. In addition, one review on the impact of ship noise on marine mammals includes a systematic literature search [ 96 ]. Two non-systematic reviews can also be cited, one about invertebrates [ 97 ] and the other about fishes [ 98 ].
Among all these bibliographic syntheses (including those from 2019), we selected those whose literature collection is based on a standardized approach (e.g. search string, database request, screening process)—which includes meta-analyses and systematic reviews/maps or similar—and whose topic is as close as possible to our systematic map (e.g. focused on noise and not on wider human pressures). We summarized the main features (topic delimitation, search strategy, number of citations) for the 12 selected evidence syntheses in Table 6 with more details in Additional file 10 .
In most cases, these reviews and meta-analyses contain far fewer articles than what we collected, which can be explained by their topic restrictions (P, E, O) as well as their search strategy (e.g. number of databases, complementary searches or not, screening criteria). In terms of topics, Shannon et al. [ 34 ] would appear to be the only standardized evidence synthesis as wide as ours (all wildlife, all sources of noise, all impacts), but the authors gathered 242 articles from 1990 to 2013. The synthesis published by Radford [ 54 ]—which, as a report, is grey literature—also provides an overview of the state of knowledge with descriptive statistics, according to a standardized method, although it focuses on non-marine organisms and it is based on 86 articles. In 2019, Kunc and Schmidt published a meta-analysis that covers all impacts of noise on animals and they collected 108 articles [ 53 ].
General comments
This map reveals that the literature on the impact of anthropogenic noise on species and ecosystems is already extensive, in that 1794 relevant articles were collected, including 1340 studies, 379 reviews and 16 meta-analyses. Studies are mainly located in North America, in particular in the United States and Canada. In Europe, the United Kingdom and the Netherlands have produced the largest numbers of articles. Australia is also active in this field.
This high volume of bibliography highlights the fact that this issue is already widely studied by scientists. The production on this topic started many years ago, around 1970, and has surged considerably since 2000. More than one hundred articles a year since 2012 are listed in our map.
This chronological pattern is quite usual and can be encountered for other topics such as light pollution [ 99 ]. It can be due to practical reasons such as better dissemination and accessibility of articles (e.g. database development), but it also certainly reflects a real increase in research activity on the topic of “noise pollution” in response to social concern for environmental issues.
The articles are mainly provided through academic sources (i.e. journal articles), but grey literature is also substantial. 461 articles included in the map (i.e. around a fourth of the map) can be grouped as ‘‘grey literature’’ (books and book chapters, reports, theses, conference objects). In particular, 36 theses from all over the world address this issue.
Regarding the population, the systematic map confirms that a very broad range of species is the topic of literature on the effects of noise pollution. Indeed, all of the 11 population classes of our coding strategy contain articles. Nevertheless, a high proportion of the map concerns mammals and, to a lesser extent birds and fishes. Among the 778 articles targeting mammals, many infrataxa are concerned (e.g. Cetacea [ 100 ], Carnivora [ 101 ], Cervidae [ 102 ], Chiroptera [ 103 ], Rodentia [ 104 ]), but the highest proportion of the articles on mammals deals with aquatic noise (500/778, 64%), which suggests that many may concern Cetacea (e.g. dolphins, whales, beluga).
The other taxonomic groups receive far less attention. Amphibians, crustaceans, mollusks, insects, reptiles and arachnids each represent 5% or less of the whole map. However, comparing these knowledge gaps to contemporary biodiversity issues, we can say, for instance, that amphibians, reptiles and invertebrates are highly threatened species [ 105 , 106 ] and noise pollution around the world is probably part of the threats [ 31 , 84 ]. These taxonomic groups are likely impacted by noise depending on the sense used. In particular, amphibians communicate extensively using sounds (i.e. chorus frogs) [ 107 ], insects demonstrate hyperacuity in directional hearing [ 108 ], reptiles (in particular snakes) and spiders can feel vibrations [ 109 , 110 , 111 , 112 ].
In terms of exposure, the map confirms that a very wide variety of anthropogenic activities generate noise and that the effects of these emissions have already been studied.
Transportation (that includes terrestrial infrastructure as well as civil aircraft and boats) is the source of noise most considered. It is closely followed by industrial sources among which high diversity is observed (e.g. pile-driving [ 113 ], seismic surveys [ 114 ], wind turbines [ 115 ], mining [ 116 ], constructions [ 117 ]). Abstract noises are in third position. This category does not necessary correspond to any precise human activities but comprises a large set of computer or machinery sounds (e.g. alarms [ 118 ], pingers [ 119 ], tones [ 120 ], pulses [ 121 ], bells [ 122 ]). Often, articles in this category do not contain many details about the source of noise. Military noise is especially studied for mammals and urban noise is significantly considered for birds (but not otherwise). Recreational noise is the least studied, however a certain diversity of sources is observable (e.g. zoo visitors [ 123 ], music festivals [ 124 ], sporst activities [ 125 ], tourists in natural habitats [ 126 ], Formula one Grand Prix racing [ 127 ], whale-watching [ 128 ]). However, urban and recreational sources of noise are important and will increase in the future because, on the one hand, urbanization is spreading all over the word and, on the other, human presence in natural habitats is also becoming more and more frequent (e.g. recreational activities in nature). For example, the expansion of Unmanned Aircraft could be a serious threat for biodiversity [ 129 ].
In terms of outcomes, the map also confirms a very wide range of impacts of noise on species and ecosystems. The most studied are the behavioural impacts involving measurements on movement [ 130 ], foraging [ 131 ], hunting [ 132 ], social behaviour [ 133 ], aversive reaction [ 134 ], etc. Biophysiology and communication are also well covered, especially the impacts on the biophysiology of mammals and fishes and on the communication birds. Biophysiological outcomes can be very diverse (e.g. hormonal response [ 135 ], heart rate [ 136 ], blood parameters [ 137 ], organ development [ 138 ]). On the other hand, the lack of literature on ecosystems, reproduction and space use is of concern. Ecosystems are a very significant aspect of biodiversity and will be increasingly integrated in public policies and scientific research, notably concerning ecosystem services in the context of global changes [ 139 , 140 ]. Reproduction and mobility of species are essential for the sustainability of their population and we already know that noise can impair them [ 141 , 142 ].
Concerning the systematic map, at the moment, we are not able to conclude whether this very rich literature provides strong evidence on impacts of anthropogenic noise on animals. Indeed, we do not know if the studies and other articles confirm or invalidate such impacts and if the studies are sufficiently robust for that purpose. However, our database highlights that a majority of studies are experimental field-based studies. This is a very good point in planning further meta-analyses or systematic reviews with the prospect of quantifying the level of impacts because these studies would probably be selected following critical analysis. For future systematic reviews/meta-analyses, we identified that the three outcomes comprising the highest number of experimental studies (which are the type of content that systematic reviews or meta-analyses would use) are: behaviour (453), biophysiology (391), communication (145).
Given the scope of our map resulting in a high number of population (P), exposure (E) and outcome (O) classes, there is a wide range of possible PEO combinations. Therefore, it is difficult to go further in this report in terms of identifying knowledge gaps and clusters and possible specific questions for future systematic reviews. At the same time, this large number of PEO combinations offers stakeholders (e.g. researchers, practitioners, decision-makers) an opportunity to gain information on the combination of interest to them.
Comparison to other evidence syntheses
It is interesting to check whether other evidence syntheses previously published have arrived at the same results, knowledge clusters and knowledge gaps as those highlighted by our map. However, given the differences in terms of methodology, topic delimitation and volume of the existing reviews, exposed in the results section, it is difficult to make such comparisons for all reviews. But we can compare our results to those from two other reviews, namely Shannon et al. [ 34 ] and Radford [ 54 ] (see Fig. 15 ).
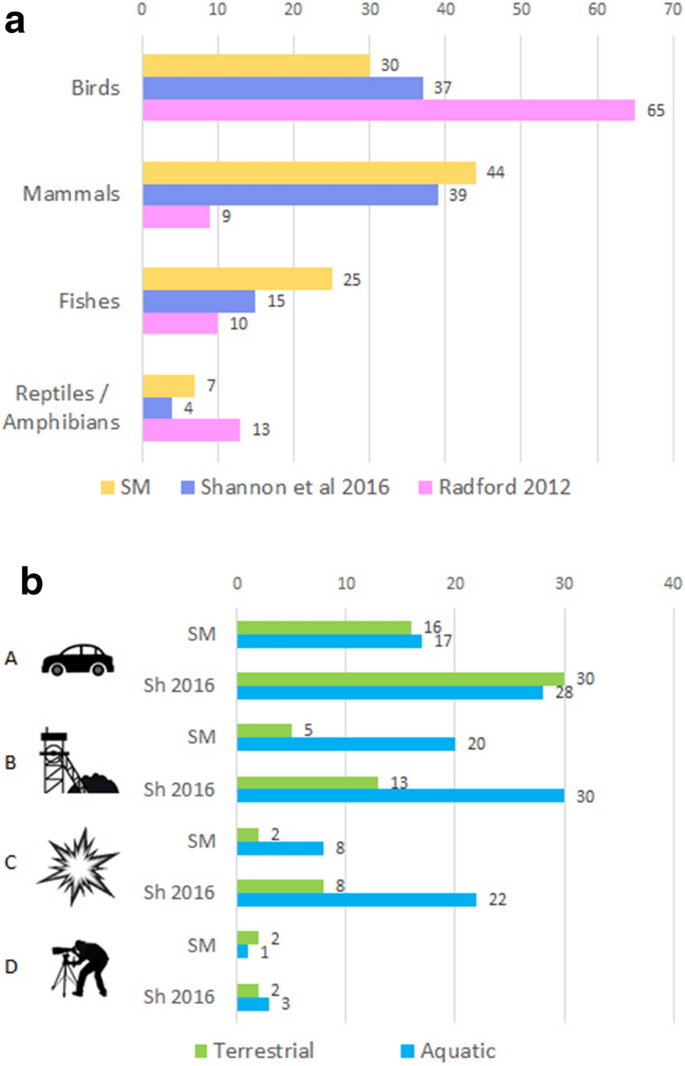
Comparison between our map results (SM) and two other standardized reviews [ 34 , 54 ] on population ( a ; top) and exposure ( b ; bottom). A = Transportation; B = Industrial; C = Military; D: Recreational
Concerning population (Fig. 15 a), mammals are the most studied species in Shannon et al. [ 34 ] (39%) as they are in our map (40%). In Radford [ 54 ], birds greatly surpass mammals (65% vs. 9%), but that can be explained by the exclusion of marine species (among which there are many mammals) in the synthesis. Fishes are more represented in our map (22%) than in the two other reviews (Shannon et al.: 15%, Radford: 10%).
Regarding exposure (Fig. 15 b), transportation is the greatest source of noise in Shannon et al. [ 34 ] for terrestrial activities (30%), similar to our map (15%). For aquatic activities, industrial noise is the exposure most frequent in our map (20%) as in Shannon et al. [ 34 ] (28%). In Radford [ 54 ], transportation noise is by far the foremost exposure (more than 75% exclusively for road and aircraft noise). These results seem to be quite consistent.
Concerning outcomes, in Shannon et al. [ 34 ], vocalization is the most frequent for terrestrial studies (44%) whereas behavioural outcomes come first in our map (19%). Behavioural is the most frequent outcome for aquatic studies in Shannon et al. [ 34 ] (more than 40%) whereas biophysiology comes first in our map (24%). Here, our results are more consistent with Radford [ 54 ], where behavioural outcomes are the most frequent (approximately 65%, compared to approximately 54% in our database).
Limitations of the systematic map
Search strategy.
We are aware that two academic databases (WOS CC and Scopus) in our search strategy is a minimum according to the CEE guidelines [ 38 ]. Nevertheless, WOS CC is the most used database in Ecology and Scopus is probably the second. Furthermore, our overall strategy includes eight bibliographic sources (see Table 4 ) and in particular three search engines. In addition, a large number of hits were exported from each of the search engines (e.g. 1000 citations for each search string on Google Scholar instead of the 300 initially expected). We also completed our search strategy with the extraction of all the bibliographic references from 37 relevant reviews. Finally, when a reference was a part of a more comprehensive article (i.e. a meeting abstract inside a proceeding with multiple abstracts), we checked whether other parts of the article could be also interesting for the map (i.e. other meeting abstracts from the same conference proceeding). We could not check systematically due to our limited resources but, nevertheless, this verification produced 36 articles that were added manually to the map.
In conclusion, although our search strategy is robust for journal articles/studies, we may have missed some relevant articles in other formats (e.g. conference papers, books, chapters). That being said, studies are the most important documentation for conducting further systematic reviews.
In addition, in light of the considerations exposed in “ Results ” and “ Discussion ” sections), our systematic map would seem to be wide-ranging and complete because it does not restrict the population, the exposure or the outcomes, contrary to the majority of reviews included in the map. The number of articles collected in the 12 systematic reviews/meta-analyses described in Table 6 shows that our map (1794 articles) constitute a very important dataset.
Full-text searching
In order to facilitate a possible additional full-text research, we have compiled a list of the unretrieved full-text texts in a dedicated Additional file 11 (Sheet 1). We could retrieve 90% of the searched full-texts which means that we had to exclude 376 articles from the map process because we could not get their full-texts. We are aware that this volume of unretrievable full-texts is not a satisfactory result, however there is no standard minimum in the CEE guidelines [ 38 ] and we did everything we could to find the full-texts. First, we benefited from different institutional accesses thanks to our map team (MNHN, CNRS, INRAE). We even performed an additional search during the Covid period when some publishers suspended their paywall. Secondly, we also asked for French and even international interlibrary loans and, when necessary, we went to the libraries to collect them. We also asked for the missing full-texts on ResearchGate. A large number of unretrieved full-texts come from the extracted relevant reviews, from Scopus and from Google Scholar (see Additional file 11 , Sheet 2 for more details on retrieved/not retrieved full-texts depending on the bibliographic sources). In the end, we could obtain some explanations for a majority of the unretrieved full-texts, i.e. 25 (7%) are available online but behind an embargo, a paywall or another access restriction, 124 (33%) are not accessible to the map team (unpublished thesis or report, unlocatable conference proceedings, only available in a print journal, etc.), 47 (13%) would be excluded during screening because of their language (according to Scopus information), 19 (5%) were requested on ResearchGate without any response.
Languages accepted at full-text screening stage
We are aware that we accepted only two languages, English and French. Nevertheless, among the 3219 screened pdf files, only 54 articles were rejected at the full-text stage because of their language. This represents less than 2%. In the end, to facilitate a possible additional screening of these full-texts, we listed them in Additional file 12 . It should also be noted that when a title or an abstract was not in English or in French, it was not rejected for this reason during the title/abstract screening, it was sent directly to abstract and/or full-text screening to check its effective language.
Coding strategy
Due to resource limitations, we were not able to perform double coding of each article by two reviewers, as requested by the CEE guidelines. We are aware that this is not a totally rigorous approach, but we anticipated it in our a priori protocol [ 36 ] because we knew that time and resources would be limited. We think that our approach did not affect coding consistency because the three coders (RS, AD, OR) followed the same coding rules and one person (RS) was present throughout the coding process to explain the rules to the other coders and to help them if necessary. In addition, at the end of the coding procedure, RS reviewed the entire map for analysis purposes.
Regarding the coding strategy, we are aware that our classification (in particular for exposure and outcome classes) is not perfect, but it is difficult to achieve a perfect solution. We decided to use published reviews such as Shannon et al. [ 34 ] or Radford [ 54 ], but different strategies exist. For example, Radford [ 54 ] split the transportation sources of noise (e.g. road, rail, boat), whereas Shannon et al. [ 34 ] grouped them in a “transportation” class. Such classes may appear too broad, but this strategy produces an initial overview of the available literature, which is certainly one of the objectives of a systematic map. As another example, the outcome class “Reproduction” was also difficult to delimit because it can include reproduction in the strictest sense (e.g. number of eggs) as well as other impacts that can influence reproduction (e.g. physiological impacts on adults in a breeding colony). In such cases, we coded the article for the different outcomes (i.e. biophysiology/reproduction).
This systematic map collated and catalogued literature dealing with the impacts of anthropogenic noise on species (excluding humans) and ecosystems. It resulted in a database composed of 1794 articles, including 1340 studies, 379 reviews and 16 meta-analyses published worldwide. Some systematic reviews and meta-analyses have already been published and were collected, however, no systematic map has yet been produced with so few topic restrictions (all wildlife, all sources of noise, all kinds of impacts) and using such a large search strategy (two databases, three search engines, etc.).
This map can be used to inform policy, provide the evidence for systematic reviews and demonstrate where more primary research is needed. It confirms that a broad range of anthropogenic activities can generate noises which may produce highly diverse impacts on a wide array of taxa. To date, some taxonomic groups (mammals, birds, fishes), types of noise (transportation, industrial, abstract) and outcomes (behavioural, biophysiological, communication) have undergone greater studies than others. Less knowledge is available on certain species (invertebrates, reptiles, amphibians), noises (recreational, urban, military) and impacts (space use, reproduction, ecosystems). Currently, this map cannot be used to determine whether the included studies demonstrate that noise does indeed produce impacts. However, it can be the starting point for more thorough syntheses of evidence. Included reviews and meta-analyses should be exploited to transfer this synthesized knowledge into operational decisions to reduce noise pollution and protect biodiversity.
Implications for policy/management
Given the volume of bibliographic data, we obviously do not face to a totally unexplored topic. But surprisingly, this rich literature on the impacts of noise pollution on biodiversity does not seem to be exploited by practitioners and decision-makers. Indeed, to date, noise pollution has been considered in terms of impacts on human health, but very little or no consideration has been given to impacts on other species and ecosystems. Two key implications emerge from this map.
First, the high volume of reviews and meta-analyses collected in this map can facilitate the immediate integration of these evidence syntheses into public policies on the national and international levels. Some reviews and the meta-analyses have quantified the level of impacts concerning the species, sources of noise and outcomes they considered. A strategy should be defined to assess the quality of these syntheses (critical appraisal) and, if reliable, transfer this already synthesized knowledge to institutional texts (e.g. regulations, guidelines, frameworks). Thanks to the exposure categorization undertaken in this map, many stakeholders and practitioners (urban planners, transport infrastructure owners, airlines and airports, military authorities, tour operators, manufacturing companies, etc.) will be able to directly identify the articles that concern their activities/structures. Such knowledge may also be useful for the European Commission, which intends to produce indicators to monitor the reduction of submarine noise pollution, as part of a new strategy for biodiversity [ 143 ].
Secondly, several knowledge clusters identified in this map may be used for new systematic reviews and meta-analyses to assess the evidence of impacts. Resources should be invested in evidence syntheses capable of exploiting the full range of the mapped literature. In particular, these analyses could determine sensitivity thresholds for guilds of species representing several natural habitats. These thresholds are essential in taking noise pollution into account for green and blue infrastructures in view of preserving and restoring quiet ecological networks. Practitioners (e.g. nature reserves and local governments) in France have started to implement this type of environmental policy and this will increase in the future [ 144 ].
Implications for research
New research programs should initiate studies on knowledge gaps, using robust experimental protocols (such as CE—Control/Exposure, BAE—Before/After/Exposure, B(D)ACE—Before(/During)/After/Control/Exposure) [ 145 , 146 , 147 , 148 ] and taking into account different types of bias [ 149 , 150 , 151 ]. In particular, studies should be started on some taxonomic groups (amphibians, reptiles and invertebrates), on certain sources of noise (recreational, military and urban) and to assess particular impacts (space use, reproduction, ecosystems) because these populations, exposures and outcomes have received little study to date. Many PEO combinations have never been studied. In addition, the findings of the current map show that research is not evenly spread worldwide, with main areas of research being in North America (United States, Canada). This finding may have an operational impact because some results may not be transposable to other contexts. Articles on further studies could also be more detailed by the authors. Indeed, some meta-data were unavailable in a significant percentage of the mapped literature. For example, the study location was unknown for 10% of the studies and approximately 1% of the articles did not indicate the source of noise or the outcome that they studied.
The map findings show that research in ecology has already addressed the issue of noise pollution. Deeper analysis is needed to assess the validity of the literature collected in this map, whether primary studies or reviews, in order to produce new syntheses and to transfer this knowledge to the applied field.
Availability of data and materials
All data, generated or analyzed during this study, are included in this published article and its addition information files.
http://www.environmentalevidence.org/guidelines/section-8 .
Dirzo R, Young HS, Galetti M, Ceballos G, Isaac NJB, Collen B. Defaunation in the anthropocene. Science. 2014;345(6195):401–6. https://doi.org/10.1126/science.1251817 .
Article CAS PubMed ADS Google Scholar
Hallmann CA, Sorg M, Jongejans E, Siepel H, Hofland N, Schwan H, et al. More than 75 percent decline over 27 years in total flying insect biomass in protected areas. PLoS ONE. 2017;12(10): e0185809. https://doi.org/10.1371/journal.pone.0185809 .
Article CAS PubMed PubMed Central Google Scholar
Ceballos G, Ehrlich PR, Dirzo R. Biological annihilation via the ongoing sixth mass extinction signaled by vertebrate population losses and declines. Proc Natl Acad Sci USA. 2017;114(30):E6089–96. https://doi.org/10.1073/pnas.1704949114 .
Article CAS PubMed PubMed Central ADS Google Scholar
Menon M, Mohanraj R. Temporal and spatial assemblages of invasive birds occupying the urban landscape and its gradient in a southern city of India. J Asia-Pac Biodivers. 2016;9(1):74–84. https://doi.org/10.1016/j.japb.2015.12.005 .
Article Google Scholar
Ripple WJ, Wolf C, Newsome TM, Galetti M, Alamgir M, Crist E, et al. World scientists’ warning to humanity: a second notice. Bioscience. 2017;67(12):1026–8. https://doi.org/10.1093/biosci/bix125 .
Mcdonald RI, Kareiva P, Forman RTT. The implications of current and future urbanization for global protected areas and biodiversity conservation. Biol Conserv. 2008;141(6):1695–703. https://doi.org/10.1016/j.biocon.2008.04.025 .
Reis E, López-Iborra GM, Pinheiro RT. Changes in bird species richness through different levels of urbanization: implications for biodiversity conservation and garden design in Central Brazil. Landsc Urban Plann. 2012;107(1):31–42. https://doi.org/10.1016/j.landurbplan.2012.04.009 .
Xu X, Xie Y, Qi K, Luo Z, Wang X. Detecting the response of bird communities and biodiversity to habitat loss and fragmentation due to urbanization. Sci Total Environ. 2018;624:1561–76. https://doi.org/10.1016/j.scitotenv.2017.12.143 .
Bennie J, Davies TW, Cruse D, Gaston KJ. Ecological effects of artificial light at night on wild plants. J Ecol. 2016;104(3):611–20. https://doi.org/10.1111/1365-2745.12551 .
Gaston KJ, Bennie J, Davies TW, Hopkins J. The ecological impacts of nighttime light pollution: a mechanistic appraisal. Biol Rev. 2013;88(4):912–27. https://doi.org/10.1111/brv.12036 .
Article PubMed Google Scholar
Warren P, Katti M, Ermann M, Brazel A. Urban bioacoustics: it’s not just noise. Anim Behav. 2006;71(3):491–502. https://doi.org/10.1016/j.anbehav.2005.07.014 .
Hempton G, Grossmann J. One square inch of silence: one man’s quest to preserve quiet. New York: Atria Books; 2010 (Reprint) .
Google Scholar
Romer H, Bailey WJ. Insect hearing in the field. Camp Biochem Physiol. 1990;97A(4):443–7. https://doi.org/10.1016/0300-9629(90)90110-E .
Sun JWC, Narins PM. Anthropogenic sounds differentially affect amphibian call rate. Biol Conserv. 2005;121(3):419–27. https://doi.org/10.1016/j.biocon.2004.05.017 .
Drolet A, Dussault C, Côté SD. Simulated drilling noise affects the space use of a large terrestrial mammal. Wildl Biol. 2016;22(6):284–93. https://doi.org/10.2981/wlb.00225 .
Bernath-Plaisted J, Koper N. Physical footprint of oil and gas infrastructure, not anthropogenic noise, reduces nesting success of some grassland songbirds. Biol Conserv. 2016;204(B):434–41. https://doi.org/10.1016/j.biocon.2016.11.002 .
Lazerte SE, Slabbekoorn H, Otter KA. Learning to cope: vocal adjustment to urban noise is correlated with prior experience in black-capped chickadees. Proc R Soc B: Biol Sci. 2016;283(1833). https://doi.org/10.1098/rspb.2016.1058 .
Cunnington GM, Fahrig L. Plasticity in the vocalizations of anurans in response to traffic noise. Acta Oecol. 2010;36(5):463–70. https://doi.org/10.1016/j.actao.2010.06.002 .
Article ADS Google Scholar
Mancera KF, Murray PJ, Lisle A, Dupont C, Faucheux F, Phillips CJC. The effects of acute exposure to mining machinery noise on the behaviour of eastern blue-tongued lizards ( Tiliqua scincoides ). Anim Welf. 2017;26(1):11–24. https://doi.org/10.7120/09627286.26.1.011 .
Amoser S, Wysocki LE, Ladich F. Noise emission during the first powerboat race in an Alpine lake and potential impact on fish communities. J Acoust Soc Am. 2004;116(6):3789–97. https://doi.org/10.1121/1.1808219 .
Article PubMed ADS Google Scholar
Shannon G, Crooks KR, Wittemyer G, Fristrup KM, Angeloni LM. Road noise causes earlier predator detection and flight response in a free-ranging mammal. Behav Ecol. 2016;27(5):1370–5. https://doi.org/10.1093/beheco/arw058 .
Bunkley JP, McClure CJW, Kawahara AY, Francis CD, Barber JR. Anthropogenic noise changes arthropod abundances. Ecol Evol. 2017;7(9):2977–85. https://doi.org/10.1002/ece3.2698 .
Article PubMed PubMed Central Google Scholar
Koper RP, Erbe C, du Preez DR, Plön S. Comparison of soundscape contributors between two neighboring southern right whale nursing areas along the South African coast. In: Fourth international conference on the effects of noise on aquatic life Dublin, Ireland, 10–16 July 2016. Proc. Mtgs. Acoust. 2016, vol. 27, 070014. https://doi.org/10.1121/2.0000303 .
Jacobsen L, Baktoft H, Jepsen N, Aarestrup K, Berg S, Skov C. Effect of boat noise and angling on lake fish behaviour: disturbance and lake fish behaviour. J Fish Biol. 2014;84(6):1768–80. https://doi.org/10.1111/jfb.12395 .
Article CAS PubMed Google Scholar
Thompson PM, Lusseau D, Barton T, Simmons D, Rusin J, Bailey H. Assessing the responses of coastal cetaceans to the construction of offshore wind turbines. Mar Pollut Bull. 2010;60(8):1200–8. https://doi.org/10.1016/j.marpolbul.2010.03.030 .
Lengagne T. Traffic noise affects communication behaviour in a breeding anuran, Hyla arborea . Biol Conserv. 2008;141(8):2023–31. https://doi.org/10.1016/j.biocon.2008.05.017 .
Vasconcelos RO, Amorim MCP, Ladich F. Effects of ship noise on the detectability of communication signals in the Lusitanian toadfish. J Exp Biol. 2007;210:2104–12. https://doi.org/10.1242/jeb.004317 .
Brown AL. Measuring the effect of aircraft noise on sea birds. Environ Int. 1990;16(4–6):587–92. https://doi.org/10.1016/0160-4120(90)90029-6 .
Mason JT, McClure CJW, Barber JR. Anthropogenic noise impairs owl hunting behavior. Biol Conserv. 2016;199:29–32. https://doi.org/10.1016/j.biocon.2016.04.009 .
Newport J, Shorthouse DJ, Manning AD. The effects of light and noise from urban development on biodiversity: implications for protected areas in Australia. Ecol Manag Restor. 2014;15(3):204–14. https://doi.org/10.1111/emr.12120 .
Morley EL, Jones G, Radford AN. The importance of invertebrates when considering the impacts of anthropogenic noise. Proc R Soc B. 2014;281(1776):20132683. https://doi.org/10.1098/rspb.2013.2683 .
Patricelli GL, Blickley JL. Avian communication in urban noise: causes and consequences of vocal adjustment. The Auk. 2006;123(3):639-649. https://doi.org/10.1642/0004-8038(2006)123[639:ACIUNC]2.0.CO;2 .
Popper AN, Hastings MC. The effects of anthropogenic sources of sound on fishes. J Fish Biol. 2009;75(3):455–89. https://doi.org/10.1111/j.1095-8649.2009.02319.x .
Shannon G, McKenna MF, Angeloni LM, Crooks KR, Fristrup KM, Brown E, et al. A synthesis of two decades of research documenting the effects of noise on wildlife: effects of anthropogenic noise on wildlife. Biol Rev. 2016;91(4):982–1005. https://doi.org/10.1111/brv.12207 .
Roca IT, Desrochers L, Giacomazzo M, Bertolo A, Bolduc P, Deschesnes R, et al. Shifting song frequencies in response to anthropogenic noise: a meta-analysis on birds and anurans. BEHECO. 2016;27(5):1269–74. https://doi.org/10.1093/beheco/arw060 .
Sordello R, Flamerie De Lachapelle F, Livoreil B, Vanpeene S. Evidence of the environmental impact of noise pollution on biodiversity: a systematic map protocol. Environ Evid. 2019;8:8. https://doi.org/10.1186/s13750-019-0146-6 .
Nugent C, Paviotti M, Bloomfield A, Kephalopoulos S, Rasmussen S, Blanes N, et al. Good practice guide on quiet areas. Luxembourg: Publications Office; 2014.
Guidelines for Authors | Environmental Evidence. http://www.environmentalevidence.org/information-for-authors . Accessed 8 June 2020.
Haddaway N, Macura B, Whaley P, Pullin A. ROSES flow diagram for systematic reviews. Version 1.0. 2018. https://figshare.com/articles/ROSES_Flow_Diagram_Version_1_0/5897389 . Accessed 8 June 2020.
Brumm H. Anthropogenic noise: implications for conservation. In: Breed MD, Moore J, editors. Encyclopedia of animal behavior. Academic Press; 2010. p. 89–93. https://doi.org/10.1016/B978-0-08-045337-8.00289-8 .
Dutilleux G, Guillard Y. Bruit urbain et faune sauvage - Synthèse bibliographique. CETE de l’Est; 2007. p. 24.
Dutilleux G, Fontaine A. Bruit routier et faune sauvage. 2015. p. 32.
Keil P, Herben T, Rosindell J, Storch D. Predictions of Taylor’s power law, density dependence and pink noise from a neutrally modeled time series. J Theor Biol. 2010;265(1):78–86. https://doi.org/10.1016/j.jtbi.2010.04.014 .
Article MathSciNet PubMed ADS Google Scholar
Calderone GJ, Butler RF. The effects of noise due to random undetected tilts and paleosecular variation on regional paleomagnetic directions. J Geophys Res. 1991;96(B3):3973–7. https://doi.org/10.1029/90JB02457 .
Rhud JL, Meagher MW. Noise stress and human pain thresholds: divergent effects in men and women. J Pain. 2001;2(1):57–64. https://doi.org/10.1054/jpai.2000.19947 .
Lipscomb DM, Son AA, Vertes D, Roettger R, Carroll J. The effect of high level sound on hearing sensitivity, cochlear sensorineuroepithelium and vasculature of the chinchilla. Acta Otolaryngol. 1977;84(1–6):44–56. https://doi.org/10.3109/00016487709123941 .
Krebs H, Macht M, Weyers P, Weijers H-G, Janke W. Effects of stressful noise on eating and non-eating behavior in rats. Appetite. 1996;26(2):193–202. https://doi.org/10.1006/appe.1996.0015 .
Kuang X. Study on environment effect of construction in Huairou District. In: Proceedings of the international conference on education, management, commerce and society, Shenyang, China. Atlantis Press; 2015. https://doi.org/10.2991/emcs-15.2015.90 .
Bee MA. Sound source segregation in grey treefrogs: spatial release from masking by the sound of a chorus. Anim Behav. 2007;74(3):549–58. https://doi.org/10.1016/j.anbehav.2006.12.012 .
Dunlop R, Noad M, Cato DH. Modification of humpback whale social sound repertoire and vocal source levels with increased noise. In: Proc. Mtgs. Acoust. 2013. ICA 2013, Montreal, Canada, 2–7 June 2013, vol. 19, 010058. https://doi.org/10.1121/1.4799213 .
Castellote M. General review of protocols and guidelines for minimizing acoustic disturbance to marine mammals from seismic surveys. J Int Wildl Law Policy. 2007;10(3–4):273–88. https://doi.org/10.1080/13880290701769262 .
Karbalaei SS, Karimi E, Naji HR, Ghasempoori SM, Hosseini SM, Abdollahi M. Investigation of the traffic noise attenuation provided by roadside green belts. Fluct Noise Lett. 2015;14(4):1550036. https://doi.org/10.1142/S0219477515500364 .
Kunc HP, Schmidt R. The effects of anthropogenic noise on animals: a meta-analysis. Biol Lett. 2019;15(11):20190649. https://doi.org/10.1098/rsbl.2019.0649 .
Radford A. The effects of noise on biodiversity (no0235)—final report for defra. 2012. p. 27.
Gomez C, Lawson JW, Wright AJ, Buren AD, Tollit D, Lesage V. A systematic review on the behavioural responses of wild marine mammals to noise: the disparity between science and policy. Can J Zool. 2016;94(12):801–19. https://doi.org/10.1139/cjz-2016-0098 .
Berger-Tal O, Wong BBM, Candolin U, Barber J. What evidence exists on the effects of anthropogenic noise on acoustic communication in animals? A systematic map protocol. Environ Evid. 2019;8:18. https://doi.org/10.1186/s13750-019-0165-3 .
Kight CR, Swaddle JP. How and why environmental noise impacts animals: an integrative, mechanistic review: environmental noise and animals. Ecol Lett. 2011;14(10):1052–61. https://doi.org/10.1111/j.1461-0248.2011.01664.x .
Dufour PA. Effects of noise on wildlife and other animals—review of research since 1971. Washington: U.S. Environmental Protection Agency; 1980. p. 102.
Mickle MF, Higgs DM. Integrating techniques: a review of the effects of anthropogenic noise on freshwater fish. Can J Fish Aquat Sci. 2018;75(9):1534–41. https://doi.org/10.1139/cjfas-2017-0245 .
Nowacek DP, Thorne LH, Johnston DW, Tyack PL. Responses of cetaceans to anthropogenic noise. Mammal Rev. 2007;37(2):81–115. https://doi.org/10.1111/j.1365-2907.2007.00104.x .
Edmonds NJ, Firmin CJ, Goldsmith D, Faulkner RC, Wood DT. A review of crustacean sensitivity to high amplitude underwater noise: data needs for effective risk assessment in relation to UK commercial species. Mar Pollut Bull. 2016;108(1–2):5–11. https://doi.org/10.1016/j.marpolbul.2016.05.006 .
Barber JR, Crooks KR, Fristrup KM. The costs of chronic noise exposure for terrestrial organisms. Trends Ecol Evol. 2010;25(3):180–9. https://doi.org/10.1016/j.tree.2009.08.002 .
Pepper CB, Nascarella MA, Kendall RJ. A review of the effects of aircraft noise on wildlife and humans, current control mechanisms, and the need for further study. Environ Manag. 2003;32(4):418–32. https://doi.org/10.1007/s00267-003-3024-4 .
Larkin RP, Pater LL, Tazik DJ. Effects of military noise on wildlife: a literature review. Report no.: USACERL Technical Report 96/21. 1996. p. 111.
Aguilar de Soto N, Gkikopoulou K, Hooker S, Isojunno S, Johnson M, Miller P, et al. From physiology to policy: a review of physiological noise effects on marine fauna with implications for mitigation. In: Fourth international conference on the effects of noise on aquatic life Dublin, Ireland 10–16 July 2016. Proc. Mtgs. Acoust. 2016, vol. 27, 040008. https://doi.org/10.1121/2.0000299 .
Brumm H, Slabbekoorn H. Acoustic communication in noise. In: Advances in the study of behavior, vol. 35. Academic Press. 2005. p. 151–209. https://doi.org/10.1016/S0065-3454(05)35004-2 .
Chapter Google Scholar
Tidau S, Briffa M. Review on behavioral impacts of aquatic noise on crustaceans. In: Fourth international conference on the effects of noise on aquatic life Dublin, Ireland 10–16 July 2016. Proc. Mtgs. Acoust. 2016, vol. 27, 010028. https://doi.org/10.1121/2.0000302 .
Nelms SE, Piniak WED, Weir CR, Godley BJ. Seismic surveys and marine turtles: an underestimated global threat? Biol Conserv. 2016;193:49–65. https://doi.org/10.1016/j.biocon.2015.10.020 .
Wang S, Wang S. Impacts of wind energy on environment: a review. Renew Sustain Energy Rev. 2015;49:437–43. https://doi.org/10.1016/j.rser.2015.04.137 .
Khairunnisa Z, Jusuf AA, Furqanita D. Differential effects of prenatal music versus noise exposure on posnatal auditory cortex development: a systematic review. J Phys: Conf Ser. 2018;1073(4):042048. https://doi.org/10.1088/1742-6596/1073/4/042048 .
Article CAS Google Scholar
Williams R, Wright AJ, Ashe E, Blight LK, Bruintjes R, Canessa R, et al. Impacts of anthropogenic noise on marine life: publication patterns, new discoveries, and future directions in research and management. Ocean Coast Manag. 2015;115:17–24. https://doi.org/10.1016/j.ocecoaman.2015.05.021 .
Cox KD, Brennan LP, Dudas SE, Juanes F. Assessing the effect of aquatic noise on fish behavior and physiology: a meta-analysis approach. In: Fourth international conference on the effects of noise on aquatic life Dublin, Ireland 10–16 July 2016. Proc. Mtgs. Acoust. 2016, vol. 27, 010024. https://doi.org/10.1121/2.0000291 .
Cox K, Brennan LP, Gerwing TG, Dudas SE, Juanes F. Sound the alarm: a meta-analysis on the effect of aquatic noise on fish behavior and physiology. Glob Change Biol. 2018;24(7):3105–16. https://doi.org/10.1111/gcb.14106 .
Cardoso GC. Nesting and acoustic ecology, but not phylogeny, influence passerine urban tolerance. Glob Change Biol. 2014;20(3):803–10. https://doi.org/10.1111/gcb.12410 .
Francis CD. Vocal traits and diet explain avian sensitivities to anthropogenic noise. Glob Change Biol. 2015;21(5):1809–20. https://doi.org/10.1111/gcb.12862 .
Cardoso GC, Hu Y, Francis CD. The comparative evidence for urban species sorting by anthropogenic noise. R Soc Open Sci. 2018;5(2):172059. https://doi.org/10.1098/rsos.172059 .
Article PubMed PubMed Central ADS Google Scholar
Fletcher JL, Busnel RG. Effects of noise on wildlife. Academic Press; 1978. https://doi.org/10.1016/B978-0-12-260550-5.X5001-9 .
Richardson WJ, Greene JCR, Malme CI, Thomson DH. Marine mammals and noise. Cambridge: Academic Press; 2013. ISBN 978-0-12-588441-9.
Brumm H, editor. Animal communication and noise. Berlin, Heidelberg: Springer; 2013. https://doi.org/10.1007/978-3-642-41494-7 .
Book Google Scholar
Popper AN, Hawkins A, editors. The effects of noise on aquatic life. New York: Springer; 2012. https://doi.org/10.1007/978-1-4419-7311-5 .
Popper AN, Hawkins A, editors. The effects of noise on aquatic life II. New York: Springer; 2016. https://doi.org/10.1007/978-1-4939-2981-8 .
Slabbekoorn H, Dooling RJ, Popper AN, Fay RR, editors. Effects of anthropogenic noise on animals. New York: Springer; 2018. https://doi.org/10.1007/978-1-4939-8574-6 .
Hawkins AD, Popper AN. Effects of man-made sound on fishes. In: Slabbekoorn H, Dooling RJ, Popper AN, Fay RR, editors. Effects of anthropogenic noise on animals. New York: Springer; 2018. p. 145–77. https://doi.org/10.1007/978-1-4939-8574-6_6 .
Simmons AM, Narins PM. Effects of anthropogenic noise on amphibians and reptiles. In: Slabbekoorn H, Dooling RJ, Popper AN, Fay RR, editors. Effects of anthropogenic noise on animals. New York: Springer; 2018. p. 179–208. https://doi.org/10.1007/978-1-4939-8574-6_7 .
Halfwerk W, Lohr B, Slabbekoorn H. Impact of man-made sound on birds and their songs. In: Slabbekoorn H, Dooling RJ, Popper AN, Fay RR, editors. Effects of anthropogenic noise on animals. New York: Springer; 2018. p. 209–42. https://doi.org/10.1007/978-1-4939-8574-6_8 .
Erbe C, Dunlop R, Dolman S. Effects of noise on marine mammals. In: Slabbekoorn H, Dooling RJ, Popper AN, Fay RR, editors. Effects of anthropogenic noise on animals. New York: Springer; 2018. p. 277–309. https://doi.org/10.1007/978-1-4939-8574-6_10 .
Borda-de-Água L, Barrientos R, Beja P, Pereira HM, editors. Railway ecology. Cham: Springer; 2017. https://doi.org/10.1007/978-3-319-57496-7 .
Gil D, Brumm H. Acoustic communication in the urban environment: patterns, mechanisms, and potential consequences of avian song adjustments. In: Gil D, Brumm H, editors. Avian urban ecology: behavioural and physiological adaptations. Oxford: Oxford University Press; 2014. https://doi.org/10.1093/acprof:osobl/9780199661572.003.0006 .
Halfwek W, Slabbekoorn H. The impact of anthropogenic noise on avian communication and fitness. In: Gil D, Brumm H, editors. Avian urban ecology: behavioural and physiological adaptations. Oxford: Oxford University Press; 2014. https://doi.org/10.1093/acprof:osobl/9780199661572.003.0007 .
Parris KM. Ecological impacts of road noise and options for mitigation. In: van der Ree R, Smith DJ, Grilo C, editors. Handbook of road ecology. Chichester: Wiley; 2015. p. 151–8. https://doi.org/10.1002/9781118568170.ch19 .
Abbott I, Berthinussen A, Stone E, Boonman M, Melber M, Altringham J. Bats and roads. In: Handbook of road ecology. 2015. p. 290–9. ISBN 978-1-118-56818-7.
Ortega CP. Effects of noise pollution on birds: a brief review of our knowledge. In: Ornithological monographs, vol. 74. 2012. p. 6–22. https://doi.org/10.1525/om.2012.74.1.6 .
Francis CD et al. Behavioral responses by two songbirds to natural-gas-well compressor noise. In: Ornithological monographs, vol. 74. 2012. p. 36–46. https://doi.org/10.1525/om.2012.74.1.36 .
Blickley JL, Patricelli GL. Potential acoustic masking of Greater Sage-Grouse ( Centrocercus urophasianus ) display components by chronic industrial noise. In: Ornithological monographs, vol. 74. 2012. p. 23–35. https://doi.org/10.1525/om.2012.74.1.23 .
Harding HR, Gordon TAC, Eastcott E, Simpson SD, Radford AN. Causes and consequences of intraspecific variation in animal responses to anthropogenic noise. Behav Ecol. 2019;30(6):1501–11. https://doi.org/10.1093/beheco/arz114 .
Erbe C, Marley SA, Schoeman RP, Smith JN, Trigg LE, Embling CB. The effects of ship noise on marine mammals—a review. Front Mar Sci. 2019;6:606. https://doi.org/10.3389/fmars.2019.00606 .
Raboin M, Elias DO. Anthropogenic noise and the bioacoustics of terrestrial invertebrates. J Exp Biol. 2019;222(12):jeb178749. https://doi.org/10.1242/jeb.178749 .
Popper AN, Hawkins AD. An overview of fish bioacoustics and the impacts of anthropogenic sounds on fishes. J Fish Biol. 2019;94(5):692–713. https://doi.org/10.1111/jfb.13948 .
Davies TW, Smyth T. Why artificial light at night should be a focus for global change research in the 21st century. Glob Change Biol. 2018;24(3):872–82. https://doi.org/10.1111/gcb.13927 .
Kendall LS, Širović A, Roth EH. Effects of construction noise on the cook inlet beluga whale (Delphinapterus leucas) vocal behavior. Can Acoust. 2013;41(3):3–14.
Chosy J, Wilson M, Santymire R. Behavioral and physiological responses in felids to exhibit construction: felid response to exhibit construction. Zoo Biol. 2014;33(4):267–74. https://doi.org/10.1002/zoo.21142 .
Espmark Y. Behaviour reactions of reindeer exposed to sonic booms. Deer. 1972;2(7):800–2.
Tressler J, Smotherman MS. Context-dependent effects of noise on echolocation pulse characteristics in free-tailed bats. J Comp Physiol A. 2009;195:923–34. https://doi.org/10.1007/s00359-009-0468-x .
Plassmann W, Kadel M. Low-frequency sensitivity in a gerbilline rodent, Pachyuromys duprasi . Brain Behav Evol. 1991;38:115–26. https://doi.org/10.1159/000114382 .
Böhm M, Collen B, Baillie JEM, Bowles P, Chanson J, Cox N, et al. The conservation status of the world’s reptiles. Biol Conserv. 2013;157:372–85. https://doi.org/10.1016/j.biocon.2012.07.015 .
Stuart SN, Chanson JS, Cox NA, Young BE, Rodrigues ASL, Fischman DL, et al. Status and trends of amphibian declines and extinctions worldwide. Science. 2004;306(5702):1783–6. https://doi.org/10.1126/science.1103538 .
Bee MA. Selective phonotaxis by male wood frogs ( Rana sylvatica ) to the sound of a chorus. Behav Ecol Sociobiol. 2007;61(6):955–66. https://doi.org/10.1007/s00265-006-0324-8 .
Römer H, Schmidt AKD. Directional hearing in insects with internally coupled ears. Biol Cybern. 2016;110(4–5):247–54. https://doi.org/10.1007/s00422-015-0672-4 .
Christensen CB, Christensen-Dalsgaard J, Brandt C, Madsen PT. Hearing with an atympanic ear: good vibration and poor sound-pressure detection in the royal python, Python regius . J Exp Biol. 2012;215(2):331–42. https://doi.org/10.1242/jeb.062539 .
Shamble PS, Menda G, Golden JR, Nitzany EI, Walden K, Beatus T, et al. Airborne acoustic perception by a jumping spider. Curr Biol. 2016;26(21):2913–20. https://doi.org/10.1016/j.cub.2016.08.041 .
Chapuis L, Kerr CC, Collin SP, Hart NS, Sanders KL. Underwater hearing in sea snakes (Hydrophiinae): first evidence of auditory evoked potential thresholds. J Exp Biol. 2019;222(14):jeb198184. https://doi.org/10.1242/jeb.198184 .
Mortimer B. A spider’s vibration landscape: adaptations to promote vibrational information transfer in orb webs. Integr Comp Biol. 2019;59(6):1636–45. https://doi.org/10.1093/icb/icz043 .
Bolle LJ, de Jong CAF, Bierman SM, van Beek PJG, van Keeken OA, Wessels PW, et al. Common sole larvae survive high levels of pile-driving sound in controlled exposure experiments. PLoS ONE. 2012;7(3):e33052. https://doi.org/10.1371/journal.pone.0033052 .
Heide-Jørgensen MP, Hansen RG, Westdal K, Reeves RR, Mosbech A. Narwhals and seismic exploration: is seismic noise increasing the risk of ice entrapments? Biol Conserv. 2013;158:50–4. https://doi.org/10.1016/j.biocon.2012.08.005 .
Larsen JK, Guillemette M. Effects of wind turbines on flight behaviour of wintering common eiders: implications for habitat use and collision risk: wind turbines and wintering common eiders. J Appl Ecol. 2007;44(3):516–22. https://doi.org/10.1111/j.1365-2664.2007.01303.x .
Alvarez-Berríos N, Campos-Cerqueira M, Hernández-Serna A, Amanda Delgado CJ, Román-Dañobeytia F, Aide TM. Impacts of small-scale gold mining on birds and anurans near the Tambopata Natural Reserve, Peru, Assessed using passive acoustic monitoring. Trop Conserv Sci. 2016;9(2):832–51. https://doi.org/10.1177/194008291600900216 .
Sutter GC, Davis SK, Skiffington JC, Keating LM, Pittaway LA. Nesting behaviour and reproductive success of sprague’s pipit ( Anthus spragueii ) and vesper sparrow ( Pooecetes gramineus ) during pipeline construction. Can Field Nat. 2016;130(2):99–109. https://doi.org/10.22621/cfn.v130i2.1833 .
Culik B, Koschinski S, Tregenza N, Ellis G. Reactions of harbor porpoises Phocoena phocoena and herring Clupea harengus to acoustic alarms. Mar Ecol Prog Ser. 2001;211:255–60. https://doi.org/10.3354/meps211255 .
Watkins WA, Schevill WE. Sperm whales ( Physeter catodon ) react to pingers. Deep Sea Res Oceanogr Abstr. 1975;22(3):123–9. https://doi.org/10.1016/0011-7471(75)90052-2 .
Finneran JJ, Carder DA, Schlundt CE, Ridgway SH. Temporary threshold shift in bottlenose dolphins ( Tursiops truncatus ) exposed to mid-frequency tonesa). J Acoust Soc Am. 2005;118(2696):10. https://doi.org/10.1121/1.2032087 .
Richard JD. Fish attraction with pulsed low-frequency sound. J Fish Res Bd Can. 1968;25(7):1441–52. https://doi.org/10.1139/f68-125 .
Fride E, Weinstock M. Alterations in behavioral and striatal dopamine asymmetries induced by prenatal stress. Pharmacol Biochem Behav. 1989;32(2):425–30. https://doi.org/10.1016/0091-3057(89)90174-3 .
Woolway EE, Goodenough AE. Effects of visitor numbers on captive European red squirrels ( Sciurus vulgaris ) and impacts on visitor experience. Zoo Biol. 2017;36(2):112–9. https://doi.org/10.1002/zoo.21357 .
Shirley MDF, Armitage VL, Barden TL, Gough M, Lurz PWW, Oatway DE, et al. Assessing the impact of a music festival on the emergence behaviour of a breeding colony of Daubenton’s bats ( Myotis daubentonii ). J Zool. 2001;254(3):367–73. https://doi.org/10.1017/S0952836901000863 .
Graham AL, Cooke SJ. The effects of noise disturbance from various recreational boating activities common to inland waters on the cardiac physiology of a freshwater fish, the largemouth bass ( Micropterus salmoides ). Aquat Conserv Mar Freshw Ecosyst. 2008;18(7):1315–24. https://doi.org/10.1002/aqc.941 .
Price M, Lill A. Behavioural responses of breeding Silver Gulls to tourist traffic. Aust Zool. 2011;35(3):810–21. https://doi.org/10.7882/AZ.2011.032 .
Payne CJ, Jessop TS, Guay P-J, Johnstone M, Feore M, Mulder RA. Population, behavioural and physiological responses of an urban population of black swans to an intense annual noise event. PLoS ONE. 2012;7:e45014. https://doi.org/10.1371/journal.pone.0045014 .
Rossi-Santos MR. Whale-watching noise effects on the behavior of humpback whales ( Megaptera novaeangliae ) in the Brazilian breeding ground. In: Fourth international conference on the effects of noise on aquatic life Dublin, Ireland 10–16 July 2016. Proc. Mtgs. Acoust. 2016, vol. 27, 040003. https://doi.org/10.1121/2.0000271 .
Mulero-Pázmány M, Jenni-Eiermann S, Strebel N, Sattler T, Negro JJ, Tablado Z. Unmanned aircraft systems as a new source of disturbance for wildlife: a systematic review. PLoS ONE. 2017;12:e0178448. https://doi.org/10.1371/journal.pone.0178448 .
Marley SA, Salgado Kent CP, Erbe C, Parnum IM. Effects of vessel traffic and underwater noise on the movement, behaviour and vocalisations of bottlenose dolphins in an urbanised estuary. Sci Rep. 2017;7:13437. https://doi.org/10.1038/s41598-017-13252-z .
Senzaki M, Yamaura Y, Francis CD, Nakamura F. Traffic noise reduces foraging efficiency in wild owls. Sci Rep. 2016;6:30602. https://doi.org/10.1038/srep30602 .
Gomes DGE, Page RA, Geipel I, Taylor RC, Ryan MJ, Halfwerk W. Bats perceptually weight prey cues across sensory systems when hunting in noise. Science. 2016;353(6305):1277–80. https://doi.org/10.1126/science.aaf7934 .
Bruintjes R, Radford AN. Context-dependent impacts of anthropogenic noise on individual and social behaviour in a cooperatively breeding fish. Anim Behav. 2013;85(6):1343–9. https://doi.org/10.1016/j.anbehav.2013.03.025 .
Phillips S. Aversive behaviour by koalas ( Phascolarctos cinereus ) during the course of a music festival in northern New South Wales, Australia. Aust Mammal. 2016;38(2):158–63. https://doi.org/10.1071/AM15006 .
Owen MA, Swaisgood RR, Czekala NM, Steinman K, Lindburg DG. Monitoring stress in captive giant pandas ( Ailuropoda melanoleuca ): behavioral and hormonal responses to ambient noise. Zoo Biol. 2004;23(2):147–64. https://doi.org/10.1002/zoo.10124 .
Borneman TE, Rose ET, Simons TR. Minimal changes in heart rate of incubating American Oystercatchers ( Haematopus palliatus ) in response to human activity. The Condor. 2014;116(3):493–503. https://doi.org/10.1650/CONDOR-14-48.1 .
Buscaino G, Filiciotto F, Buffa G, Bellante A, Stefano VD, Assenza A, et al. Impact of an acoustic stimulus on the motility and blood parameters of European sea bass ( Dicentrarchus labrax L.) and gilthead sea bream ( Sparus aurata L.). Mar Environ Res. 2010;69(3):136–42. https://doi.org/10.1016/j.marenvres.2009.09.004 .
Li ZZ, Burke WH. Influence of 12 hours of sound stimuli on gonad development and plasma luteinizing hormone in Japanese Quail ( Cotumix coturnix japonica ) exposed to 6 hours of daily light. Poult Sci. 1987;66(6):1045–52. https://doi.org/10.3382/ps.0661045 .
Malhi Y, Franklin J, Seddon N, Solan M, Turner MG, Field CB, et al. Climate change and ecosystems: threats, opportunities and solutions. Phil Trans R Soc B. 2020;375(1794):20190104. https://doi.org/10.1098/rstb.2019.0104 .
Van der Biest K, Meire P, Schellekens T, D’hondt B, Bonte D, Vanagt T, et al. Aligning biodiversity conservation and ecosystem services in spatial planning: focus on ecosystem processes. Sci Tot Environ. 2020;712:136350. https://doi.org/10.1016/j.scitotenv.2019.136350 .
Chen HL, Koprowski JL. Animal occurrence and space use change in the landscape of anthropogenic noise. Biol Conserv. 2015;192:315–22. https://doi.org/10.1016/j.biocon.2015.10.003 .
Nabi G, McLaughlin RW, Hao Y, Wang K, Zeng X, Khan S, et al. The possible effects of anthropogenic acoustic pollution on marine mammals’ reproduction: an emerging threat to animal extinction. Environ Sci Pollut Res. 2018;25:19338–45. https://doi.org/10.1007/s11356-018-2208-7 .
European Commission. Communication from the Commission to the European Parliament, the Council, the European Economic and Social Committee and the Committee of the Regions. EU Biodiversity Strategy for 2030. Bringing nature back into our lives. COM(2020) 380 final, Brussels, 20 May 20 2020. https://ec.europa.eu/info/sites/info/files/communication-annex-eu-biodiversity-strategy-2030_en.pdf .
Bougnec AL. Mémoire de première année de Master Gestion territoriale du développement durable. 2018. p. 79.
Tyack P, Gordon J, Thompson D. Controlled exposure experiments to determine the effects of noise on marine mammals. Mar Technol Soc J. 2003;37(4):41–53. https://doi.org/10.4031/002533203787537087 .
Smokorowski KE, Randall RG. Cautions on using the before-after-control-impact design in environmental effects monitoring programs. FACETS. 2017;2:212–32. https://doi.org/10.1139/facets-2016-0058 .
De Palma A, Sanchez-Ortiz K, Martin PA, Chadwick A, Gilbert G, Bates AE, et al. Challenges with inferring how land-use affects terrestrial biodiversity: study design, time, space and synthesis. In: Advances in ecological research. Elsevier; 2018. p. 163–99. https://doi.org/10.1016/bs.aecr.2017.12.004 .
Christie AP, Amano T, Martin PA, Shackelford GE, Simmons BI, Sutherland WJ. Simple study designs in ecology produce inaccurate estimates of biodiversity responses. J Appl Ecol. 2019;56(12):2742–54. https://doi.org/10.1111/1365-2664.13499 .
Faulkner RC, Farcas A, Merchant ND. Guiding principles for assessing the impact of underwater noise. J Appl Ecol. 2018;55(6):2531–6. https://doi.org/10.1111/1365-2664.13161 .
Wade L, Whitehead H, Weilgart L. Conflict of interest in research on anthropogenic noise and marine mammals: does funding bias conclusions? Mar Policy. 2010;34(2):320–7. https://doi.org/10.1016/j.marpol.2009.08.009 .
Stebbins WG, Moody DB, Serafin JV. Some principal issues in the analysis of noise effects on hearing in experimental animals. Am J Otolaryngol. 1982;3(4):295–304. https://doi.org/10.1016/S0196-0709(82)80069-0 .
Download references
Acknowledgements
The map team thanks:
Dakis-Yaoba Ouédraogo (MNHN) and Yorick Reyjol (OFB) for providing comments on earlier versions of the manuscript;
Marc Morvan, Magali Morvan and Benoît Pichet from the library of the National Museum of natural History for their help during the pdf search;
All the institutions that transmitted full-texts to us during the pdf search, namely the library of the “Arts-et-Métiers” (Isabelle FERAL), the library of the “Ecole de Médecine” (Isabelle Beaulande), the library of the “Maison des Sciences de l’Homme” (Amélie Saint-Marc), the library of the “École Polytechnique” (Claire Vandermeersch), the library of “Sorbonne Université” (Isabelle Russo and Peggy Bassié), the library of “Paris 13 Villetaneuse”, ZeFactory ARTELIA (Magalie Rambaudi);
all the organizations that relayed our call for literature through their websites or mailing lists, namely the “Centre de ressources Trame verte et bleue”, the IENE, the ITTECOP;
everyone who transmitted literature to us during the call, namely Vital Azambourg (MNHN), Ludivine Boursier (FRB), Fabien Claireau (MNHN), Patricia Detry (CEREMA), Cindy Fournier (MNHN), Philippe Goulletquer (IFREMER), Aurelie Goutte, Anne Guerrero (SNCF Réseau), Eric Guinard (CEREMA), Heinrich Reck, Antonin Le Bougnec (PNR Morbihan), Barbara Livoreil (FRB), Sylvain Moulherat (TerrOïko), Dakis-Yaoba Ouédraogo (MNHN), Marc Thauront (Ecosphère), Dennis Wansink (BUWA);
Barbara Livoreil (FRB) for her help with the protocol of this map;
Cary Bartsch for his proofreading and corrections concerning the English language.
This research was undertaken as current work of UMS Patrimoine Naturel, a joint research unit funded by the French Biodiversity Agency (OFB), the National Scientific Research Center (CNRS) and the National Museum of Natural History (MNHN), on behalf of the French Ecology Ministry.
Author information
Authors and affiliations.
UMS Patrimoine Naturel (PATRINAT), OFB-CNRS-MNHN, 36 rue Geoffroy-Saint-Hilaire CP41, 75005, Paris, France
Romain Sordello, Ophélie Ratel & Alexis Dambry
Université de Bordeaux, 33400, Talence, France
Frédérique Flamerie De Lachapelle
Direction générale déléguée aux collections - Bibliothèque centrale - Service Recherche, enseignement, expertise, Museum national d’Histoire naturelle (MNHN), 75005, Paris, France
Clément Leger
Institut national de recherche pour l’agriculture, l’alimentation et l’environnement (INRAE PACA Centre d’Aix-en-Provence), 13182, Aix-en-Provence, France
Sylvie Vanpeene
You can also search for this author in PubMed Google Scholar
Contributions
RS originated the idea of the systematic map and was the scientific coordinator of the map. RS conducted the first scoping stage. FF participated in the search strategy. RS, SV and AD screened the articles. RS searched the full-texts with help from FF and CL. OR, AD and RS extracted the metadata. RS analysed, interpreted and discussed the results, helped by the rest of the team. RS wrote the draft of the manuscript and the rest of the team contributed to it. All authors read and approved the final manuscript.
Corresponding author
Correspondence to Romain Sordello .
Ethics declarations
Ethics approval and consent to participate.
Not applicable.
Consent for publication
Competing interest.
The authors declare that they have no competing interests.
Additional information
Publisher's note.
Springer Nature remains neutral with regard to jurisdictional claims in published maps and institutional affiliations.
Supplementary information
Additional file 1..
ROSES form.
Additional file 2.
Search strategy.
Additional file 3.
Key reviews from which bibliographic references were extracted.
Additional file 4.
Comprehensiveness of databases and search engines.
Additional file 5.
Detailed screening process.
Additional file 6.
Full flow diagram.
Additional file 7.
Inclusion/exclusion decisions during the three screening stages and extraction of rejected full-texts.
Additional file 8.
Accepted full-texts published in 2019–2020.
Additional file 9.
Systematic map database.
Additional file 10.
Information on standardized evidence syntheses.
Additional file 11.
List and statistics on missing full-texts.
Additional file 12.
Rejected full-texts (language exclusion).
Rights and permissions
Open Access This article is licensed under a Creative Commons Attribution 4.0 International License, which permits use, sharing, adaptation, distribution and reproduction in any medium or format, as long as you give appropriate credit to the original author(s) and the source, provide a link to the Creative Commons licence, and indicate if changes were made. The images or other third party material in this article are included in the article's Creative Commons licence, unless indicated otherwise in a credit line to the material. If material is not included in the article's Creative Commons licence and your intended use is not permitted by statutory regulation or exceeds the permitted use, you will need to obtain permission directly from the copyright holder. To view a copy of this licence, visit http://creativecommons.org/licenses/by/4.0/ . The Creative Commons Public Domain Dedication waiver ( http://creativecommons.org/publicdomain/zero/1.0/ ) applies to the data made available in this article, unless otherwise stated in a credit line to the data.
Reprints and permissions
About this article
Cite this article.
Sordello, R., Ratel, O., Flamerie De Lachapelle, F. et al. Evidence of the impact of noise pollution on biodiversity: a systematic map. Environ Evid 9 , 20 (2020). https://doi.org/10.1186/s13750-020-00202-y
Download citation
Received : 17 March 2020
Accepted : 25 August 2020
Published : 11 September 2020
DOI : https://doi.org/10.1186/s13750-020-00202-y
Share this article
Anyone you share the following link with will be able to read this content:
Sorry, a shareable link is not currently available for this article.
Provided by the Springer Nature SharedIt content-sharing initiative
- Man-made sounds
- Anthropogenic sounds
- Auditory masking
- Acoustic stimuli
- Urbanization
- Species loss
- Natural habitats
Environmental Evidence
ISSN: 2047-2382
- Submission enquiries: Access here and click Contact Us
- General enquiries: [email protected]
Thank you for visiting nature.com. You are using a browser version with limited support for CSS. To obtain the best experience, we recommend you use a more up to date browser (or turn off compatibility mode in Internet Explorer). In the meantime, to ensure continued support, we are displaying the site without styles and JavaScript.
- View all journals
- My Account Login
- Explore content
- About the journal
- Publish with us
- Sign up for alerts
- Open access
- Published: 09 February 2023
The applicability of species sensitivity distributions to the development of generic doses for phytosanitary irradiation
- Cory Penca 1 ,
- Andrea L. Beam 1 &
- Woodward D. Bailey 1
Scientific Reports volume 13 , Article number: 2358 ( 2023 ) Cite this article
719 Accesses
1 Citations
Metrics details
- Invasive species
Ionizing radiation is used as a phytosanitary treatment to prevent the introduction of pests through trade. Generic doses are a valuable means to increase the number of pest-commodity combinations that can be treated using phytosanitary irradiation. Generic doses allow for the treatment of the entire taxa for which the dose has been approved, allowing for the treatment of untested species. As such, the approval of a generic dose requires substantial supporting data and careful consideration of the risks involved. We adopt the Species Sensitivity Distribution (SSD) framework, already in widespread use in the field of ecotoxicology and environmental risk assessment, to evaluate generic doses for phytosanitary irradiation treatments. Parametric SSDs for Curculionidae and Tephritidae were developed using existing data on efficacious phytosanitary irradiation treatments. The resulting SSDs provided estimates of the taxa coverage expected by the generic dose, along with the margin of uncertainty. The SSD analysis lends support to the existing 150 Gy generic dose for Tephritidae and a proposed 175 Gy generic dose for Curculionidae. The quantitative estimates of risk produced by the SSD approach can be a valuable tool for phytosanitary rule making, improving the process for generic dose development and approval.
Similar content being viewed by others
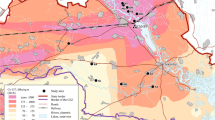
Assessment of exposure to ionizing radiation in Chernobyl tree frogs (Hyla orientalis)
Pablo Burraco, Clément Car, … Germán Orizaola
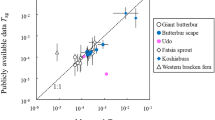
Aggregated transfer factor of 137Cs in edible wild plants and its time dependence after the Fukushima Dai-ichi nuclear accident
Momo Takada, Tetsuo Yasutaka, … Keiko Tagami
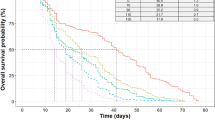
X-rays are as effective as gamma-rays for the sterilization of Glossina palpalis gambiensis Vanderplank, 1911 (Diptera: Glossinidae) for use in the sterile insect technique
Bénéwendé Aristide Kaboré, Arooj Nawaj, … Chantel J. de Beer
Introduction
The introduction of invasive species through global trade represents one of the most significant threats to global biodiversity and ecosystem services and is responsible for billions of dollars in economic losses to the agricultural sector through direct crop losses, increased management costs and loss of market access 1 , 2 , 3 , 4 , 5 . Phytosanitary treatments are an invaluable means of preventing the introduction of invasive species while facilitating global trade 6 . For established treatment technologies, such as methyl bromide fumigation, the acceptance of current phytosanitary treatment schedules is based on a combination of supporting research and a history of effective use. For newer treatment technologies, which lack a track record of efficacy, a substantial body of supporting research is required before acceptance by a National Plant Protection Organization (NPPO) or appropriate regulatory authority. Supporting research includes strong evidence of species-specific treatment efficacy, and in many cases the approval of the new treatment will be limited to the specific pest-commodity combinations where testing has occurred. For a single-species treatment schedule to be approved most regulatory authorities require a large sample size be tested without treatment failure. Historically this could require up to 93,613 individual insects being treated, as is the case for proving at least probit-9 level efficacy 7 . Arguments have been made that probit-9 criteria may be excessive for some pests, and methods for approving treatments that require smaller sample sizes have been proposed 8 , 9 , 10 . Nonetheless, widespread adoption of newer treatment technologies is limited in part by the lack of approved treatment schedules caused by the difficulties associated with building a body of supporting research to defend their use. Therefore, older technologies like methyl bromide fumigation are still widely used and their replacement has been slow.
Generic doses have been used by NPPOs, including the United States Department of Agriculture, Animal and Plant Health Inspection Service’s Plant Protection and Quarantine program (USDA-APHIS-PPQ), to increase the number of pest-commodity combinations for which a treatment can be used. A generic dose is a treatment schedule considered by researchers and/or regulatory authorities to be effective at mitigating all members of a specified taxon. In essence, generic doses increase the potential customer base for the phytosanitary treatment, providing an economic incentive for the wider adoption of the treatment technology. Generic doses offer an additional advantage when a new pest species is detected in a country. Such a discovery could disrupt trade until research is conducted to determine a treatment schedule for the new pest. However, if a generic treatment is available which covers the new pest, trade can continue with minimal or no disruption 11 .
The current method for establishing a generic dose for a particular taxon typically involves reviewing the literature and identifying relevant studies where the treatment has been applied to the taxon in question. A subjective judgement is then made as to whether enough members of the taxon have been tested and then a treatment dose at or near the dose required to control the most tolerant member of the taxon is selected to serve as the generic dose. Whether the generic dose is likely to cover species not represented by the literature review is unknown and criteria are not established for the number of species in a taxon which must be studied before a generic dose can be approved for that group. In general, if data are present for the majority of the most economically important or quarantine significant species then there is confidence that the generic dose will be secure enough for phytosanitary use. This general approach has been used in proposing generic irradiation treatments at the genus level (i.e. Anastrepha ), the family level, as in Tephritidae 12 , 13 and Tortricidae 14 , the level of order for Lepidoptera 15 , and broadly for the class Insecta excluding pupal and adult Lepidoptera 16 . Generic doses for these taxa are included in the APHIS-PPQ Treatment Manual and the generic doses for Anastrepha , Tephritidae and Tortricidae have been accepted by the International Plant Protection Convention’s Commission for Phytosanitary Measures and have been adopted under ISPM 28: Phytosanitary treatments for regulated pests 17 , 18 .
A major drawback to current ‘subjective’ approaches to generic dose setting is that there is a lack of information on what portion of the taxa might require a dose higher than the generic dose. Having an estimate of the taxonomic coverage of the generic dose will provide policy makers with a quantification of the potential risk that a member of the taxa will not be controlled by the treatment. A possible means to achieve a quantitative estimate of the taxonomic coverage of a generic dose is via the species sensitivity distribution (SSD) method, a widespread approach in ecotoxicology 19 . The SSD method looks at the distribution of toxicity values for multiple species, and after fitting these values to an appropriate distribution (as in the parametric approach), estimates the percentile of species affected at varying doses/concentrations from the resulting cumulative distribution function 20 . Species sensitivity distributions have been used to set environmental limits for a variety of toxicants, most frequently in aquatic environments. The SSD approach has been adopted in various forms by environmental regulatory agencies in numerous countries 19 , 21 . The United States Environmental Protection Agency has developed a web-accessible “SSD Toolbox” to assist with conducting and interpreting SSD analysis for environmental toxicants (EPA 2020), whereas Australia and New Zealand have co-developed a software tool called “Burrlioz” for similar purposes 22 . A key advantage of the SSD approach is the ability to extrapolate beyond the available data and provide estimates of uncertainty 23 . We introduce the SSD method as a tool for evaluating generic doses for phytosanitary treatments with a demonstration using reported phytosanitary irradiation doses for the dipteran family Tephritidae and the coleopteran family Curculionidae. We conducted the SSD approach by using available data for Curculionidae and Tephritidae, along with an interval-censored SSD to adjust for differences in data quality presented in the Curculionidae source studies. We address the challenges to the successful application of the SSD approach, referring to the body of ecotoxicology literature that has been generated in an attempt to address these challenges. Specifically, we address concerns regarding data quality in the source studies and the challenges of correctly interpreting the results of an SSD analysis when setting the minimum dose in a phytosanitary treatment schedule. Lastly, we provide recommendations for future phytosanitary irradiation research that accommodates and incorporates the use of the SSD approach to generic dose setting for phytosanitary treatments.
Curculionidae crude SSD
A total of 28 species of Curculionidae from 20 genera were identified where studies on the irradiation dose required for prevention of F1 development to adults were present in the literature (Table 1 ). Our Curculionidae literature review expands on those of Hallman 24 (14 species) and Follett 25 (15 species) who have proposed a generic dose of 150 Gy for this family. The highest reported dose in our literature review was 250 Gy, reported for Naupactus xanthographus. This dose appeared to be arbitrarily selected (no doses below 250 Gy were tested) and was identified as an extreme outlier (Cook’s distance greater than 4/ n ). This dose was removed from the dataset used for the calculation of the SSD.
The Weibull distribution showed the best fit for the Curculionidae data based on AIC, however the differences between distributions were small (Table 2 ). The resulting SSD curve for Curculionidae is presented in Fig. 1 . The GD90 and GD95 estimates (i.e., the generic doses predicted to control 90% and 95% of the taxa) for the Weibull distribution were both lower than 175 Gy, with the 90% CI for the GD90 estimate falling entirely below 175 Gy for the Weibull distribution (Fig. 2 ). At a dose of 175 Gy the estimated species coverage was 95.4% (90% CI: 90.2–99.4%). In comparison, the proposed 150 Gy generic dose provides an estimated species coverage of 89.2% (90% CI: 82.0–96.8%).
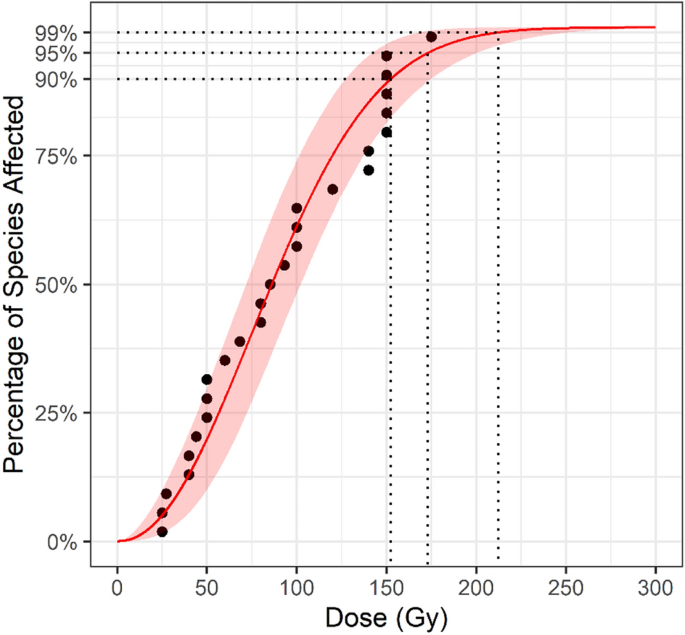
SSD Curve for Curculionidae fitted to the Weibull distribution with the shaded area covering the 5–95% confidence interval.
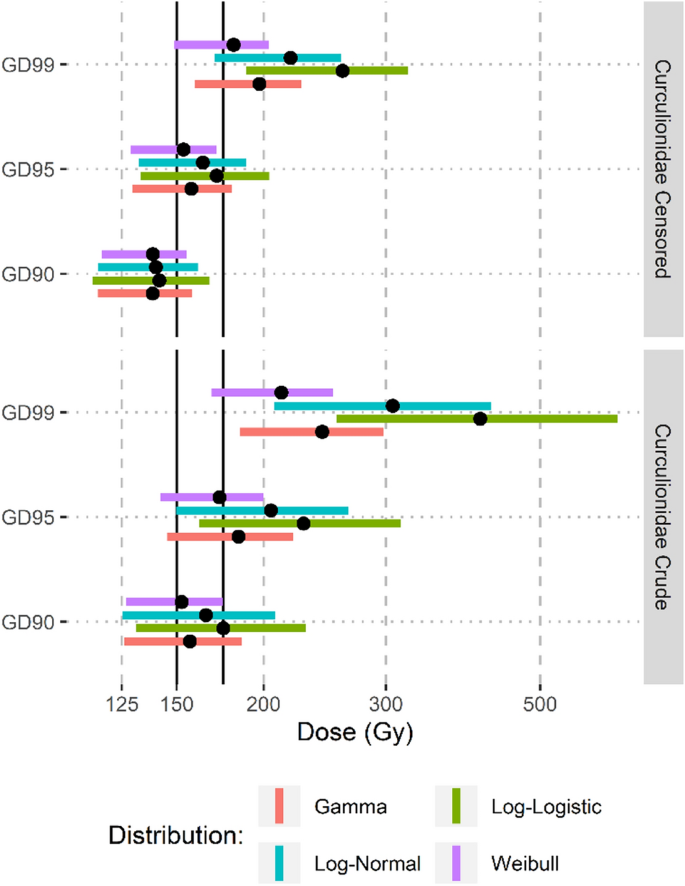
Point estimates and the lower 5% to upper 95% confidence intervals (colored bars) for the generic irradiation dose required to obtain 90, 95 and 99 percent coverage of the family Curculionidae.
Curculionidae Interval Censored SSD
The lognormal distribution provided the best fit for the interval censored dataset. The resulting SSD curve from the interval censored data was steeper than the SSD curve resulting from the crude analysis (Fig. 3 ). Estimated coverage at the range of interest was increased when using the interval censored SSD and the point estimates converged around 190 Gy (Fig. 4 ). At 175 Gy, the estimated species coverage for the interval censored SSD was 96.4% (90% CI: 93.0–99.3%) while the proposed 150 Gy generic dose provides an estimated species coverage of 92.5% (90%CI: 87.1–98.0%). The interval censored – lognormal estimate for the GD90 was 140.08 Gy (90% CI: 115.74–161.02), for the GD95 the estimate was 163.59 Gy (90% CI: 132.37—189.04) and for the GD99 the estimate was 218.84 Gy (90% CI: 170.10–258.54) (Fig. 2 ). When interval-censored estimates were compared to crude estimates from the same distributions the interval-censored estimates were lower, ranging from a 13- 33 Gy difference at the GD90 coverage level to a 31- 150 Gy difference at the GD99 coverage level, with the largest differences between crude and interval censored estimates occurring in the log-logistic SSD (Fig. 2 ).
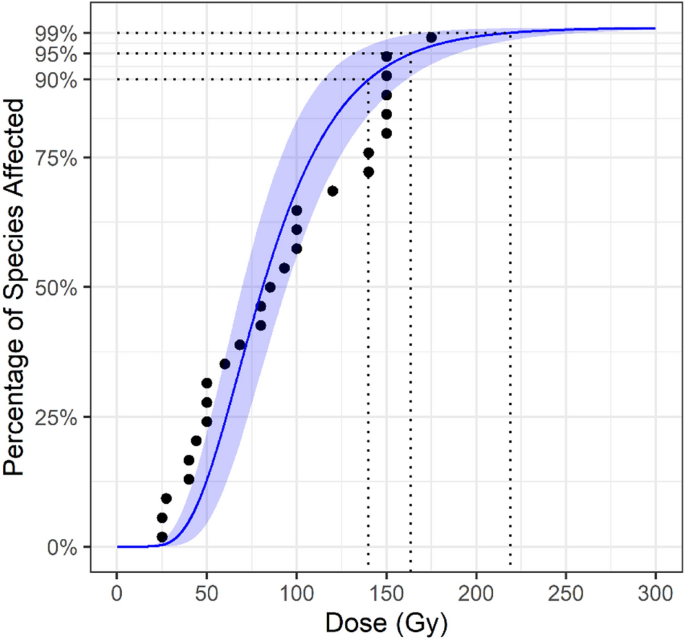
SSD Curve for Curculionidae using interval-censored data with the shaded area covering the 5–95% confidence interval. Points are fitted to the log-normal distribution.
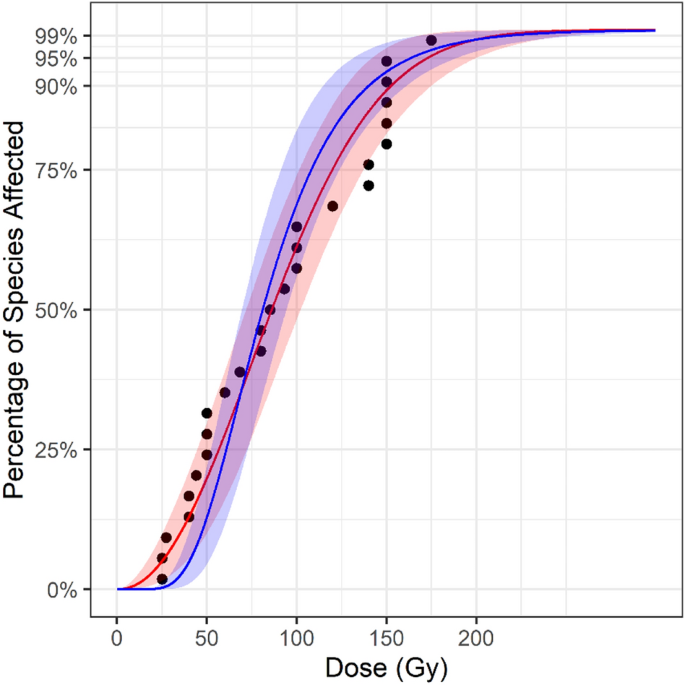
Comparison of the Crude SSD (red) and Interval Censored SSD (blue) for Curculionidae.
Tephritidae crude SSD
A total of 15 species of Tephritidae were identified where research has been conducted on the ability of irradiation to prevent late instar larvae from developing to adults. For several species multiple studies had been conducted and were combined into a single dose for analysis. Table 3 presents the combined doses used to generate SSD curves.
The gamma distribution provided the best fit for the Tephritidae data (Table 2 ). The resulting SSD curve is presented in Fig. 5 . The GD90, GD95 and GD99 were estimated at 113.88 (90% CI: 84.07–141.86), 133.08 (90% CI: 96.18–168.13), 174.30 (90% CI: 120.21–225.19) respectively (Fig. 6 ). The U.S. Department of Agriculture (USDA)-approved generic dose of 150 Gy was estimated to cover 97.4% of species in Tephritidae (90 %CI: 92.1 – 99.9).
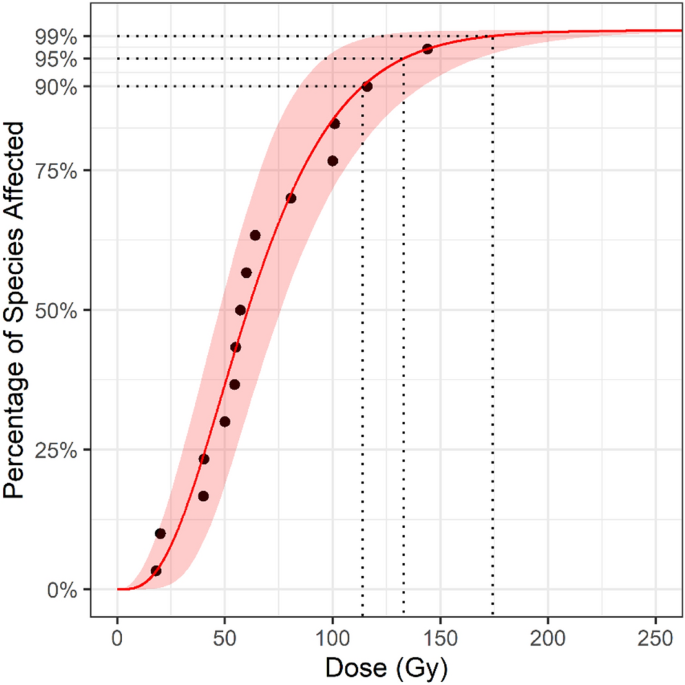
SSD Curve for Tephritidae with the shaded area covering the 5–95% confidence interval. Points are fitted to the gamma distribution.
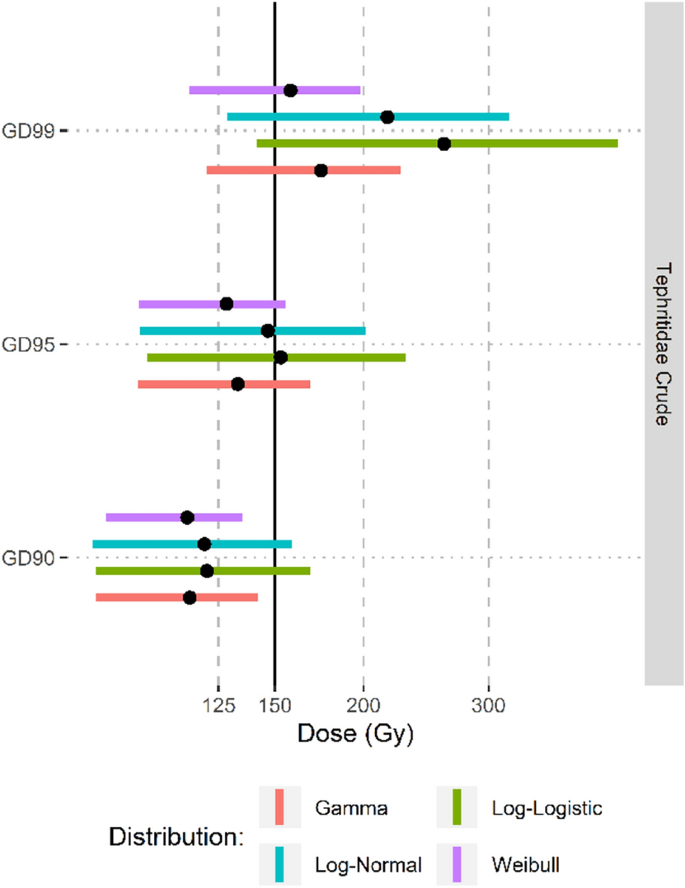
Point estimates and the lower 5% to upper 95% confidence intervals (colored bars) for the generic irradiation dose required to obtain 90, 95 and 99 percent coverage of the family Tephritidae.
Tephritidae small sample size bias correction
When the Cox-Snell method for small sample size bias correction was applied to the Tephritidae dataset, the resulting SSD curve was shifted leftward with the estimates for the GD90, GD95 and GD99 values being 12.29, 12.93, and 13.93 Gy lower, respectively, than the estimates from the gamma distributed crude SSD (Fig. 7 ). At the 150 Gy approved generic dose the sample size bias corrected SSD provided 1.11% greater coverage than the gamma distributed crude SSD.
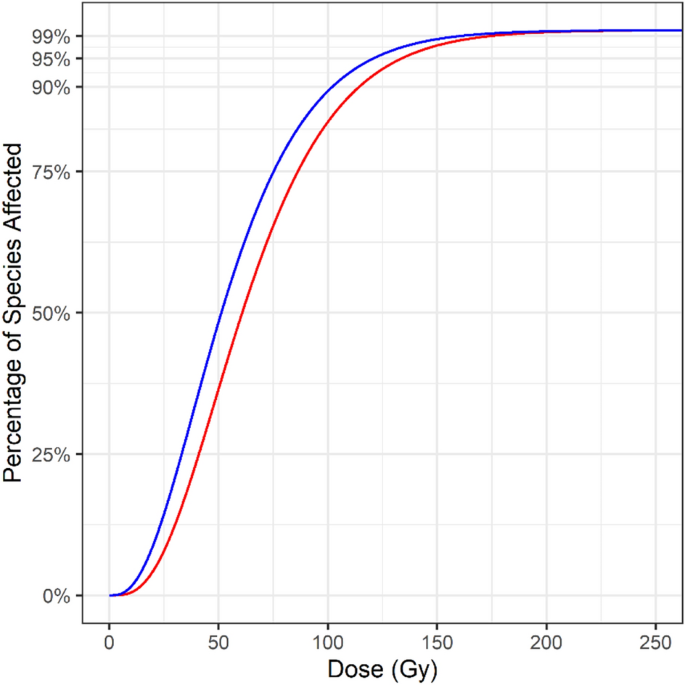
Comparison between the small sample size bias-corrected SSD (blue) and the crude SSD (red). The leftward shift indicates that increasing the number of Tephritidae species used in the SSD model will result in a reduction in the required generic dose.
The present study provides an example of how species sensitivity distributions can be applied to generic doses for phytosanitary treatments, providing a valuable quantification of the expected taxonomic coverage of the generic dose. This technique could be widely applicable and assist the international phytosanitary community in making decisions about adopting new phytosanitary treatments. The desired level of coverage necessary for a generic phytosanitary irradiation treatment is not resolved and may ultimately be determined be each country based on an assessment of acceptable level of risk. In this work we presented results at 3 levels of coverage (GD90, GD95, GD99), however the actual level of coverage required should be based on a consideration of various risk factors associated with the taxa and commodity of interest, including infestation rates, consignment sizes, establishment probabilities and the impact of establishment of the major pests within the taxa. Coverage greater than 95% (GD95) was obtained for Tephritidae at the current APHIS-approved generic dose of 150 Gy, and for Curculionidae at the proposed generic dose of 175 Gy. Whether coverage at the GD95 level is sufficient requires careful consideration. In the case of both Tephritidae and Curculionidae the generic doses were developed using data that included several of the most important pest species in each taxa and the recommended doses are above the required dose for the most radiotolerant of these pest species. Furthermore, the majority of species in both taxa are rarely (or never) encountered in trade and are not considered important agricultural or environmental pests. Thus, it is unlikely that a species in the < 5% of the taxa not covered by the generic dose will be consequential in the phytosanitary treatment context.
In practice phytosanitary irradiation treatments do not deliver precise doses; rather a range of absorbed doses will be experienced, spanning the minimum dose (Dmin) up to the maximum dose (Dmax). The dose uniformity ratio for an irradiation treatment is equal to Dmax/Dmin and can vary between treatment operators depending on commodity density, process configuration and irradiation source. In order for a phytosanitary irradiation treatment to be considered successful the measured Dmin value must not fall below the dose required in the approved treatment schedule. The actual dose received by a pest may be 20–100% greater than the required dose depending on the DUR for that treatment. The increased dose absorbed due to the DUR provides an additional level of protection beyond what is predicted by the SSD method, thus the estimates given by the SSD method can be considered conservative in practice. If the required treatment dose to obtain sufficient coverage is not feasible due to damage to the commodity, then phytosanitary irradiation may not be an appropriate treatment for that commodity. It may also prove desirable to increase the generic dose until the slope of the SSD falls below a value m (i.e., for every 1 Gy increase in dose a m % increase in coverage is obtained). This approach implies a trade-off between the cost of increasing the minimum dose requirement and the reduction of risk obtained from increasing taxa coverage. The nature of this relationship, and the value for m, should be defined. Following this method when an arbitrary m value of 0.1% is used, then the suggested generic dose for Curculionidae derived from the censored SSD would be 177 Gy, beyond which the gain in coverage per unit increase in dose is less than 0.1%. A dose of 177 Gy is estimated to be effective to control 96.6% of species in Curculionidae.
Data quality plays an important role in producing accurate estimates from SSD models. While a number of factors influence the perceived quality of the estimates produced by the studies used to develop the SSD model, in phytosanitary irradiation research two primary factors help differentiate data quality. The first is the presence of testing at multiple doses below the recommended dose. Under an ideal design these results can be analyzed by regression to produce an estimate at a given level of efficacy along with a confidence interval for that estimate. Even in studies where a dose–response analysis was not conducted, having multiple doses tested below the recommended treatment dose helps limit the magnitude of an overestimate. The second data quality factor is the number of specimens tested at the recommended dose. In phytosanitary research this is referred to as confirmatory testing, and the current standard is to test upwards of 30,000 (probit 8.72) to 93,613 (probit 9) individuals with no survivors. Increasing the number of individuals tested at the recommended dose will prevent underestimating the phytosanitary dose. For example, if 50 Gy was the probit 6-equivalent dose (84.13% efficacy at the 95% confidence level), and only 12 specimens were tested, the probability of observing no survivors is approximately 12.5%, whereas if 200 specimens were tested, the probability of observing no survivors is less than 0.00003%. The efficacy at a given dose is not known a priori , however as efficacy increases with dose, it follows that higher values of n at lower doses will prevent misleading observations of 100% efficacy at doses below the true phytosanitary dose. When treatment efficacy requirements are determined using a risk-based approach efficacy levels below 99.99% / probit-8.72 can be considered sufficient for phytosanitary treatments, as may occur in cases where the initial infestation rate is low due to poor host status of the commodity or effective management of the pest at the place of production 10 , 50 . In such cases an SSD-derived generic dose that aims to achieve 99.99% or greater efficacy for each species in the taxa may result in “overkill”, wherein the treatment is in excess of what would be required to reach the desired risk-based criteria for efficacy.
Our conclusion that a generic dose of 175 Gy can be expected to control 96.4% of Curculionidae contains several important caveats. The treatment end points included studies where F1 mortality occurred in the egg stage and also studies where F1 mortality occurred after eclosion but none of the F1 generation developed to the adult stage. The doses required to prevent egg hatch and the doses required to prevent F1 larvae from reaching the adult stage are not equivalent, and this complicates direct comparisons. This highlights the importance of using consistent endpoints in generic dose research. We believe the source data includes an inherent bias towards conservative (higher) doses. This will result in shifting the SSD curve to the right, resulting in underestimate coverage at proposed doses. Underestimating the required dose, as occurs when too few specimens are tested, can also impact our model by increasing the variance around the mean. Our source data included several reported 40 Gy doses, conducted with only 12 insects 48 , 49 . These data points likely do not represent a true phytosanitary dose. The shifting of the curve at the extremes can influence distribution selected for the SSD model and ultimately the dose estimates for GD95. When the issue of data quality and variability in the confidence in the reported doses was addressed using interval censored data, we observed a downward shift in the doses required to obtain taxonomic coverage at GD90, 95 and 99.
A key assumption when using SSDs is that the source data is unbiased for the group being observed. In our example, the species selected from Curculionidae and Tephritidae are decidedly biased; they are largely the economically important species detected in trade. For example, 6 of the 10 most frequently intercepted Curculionidae genera reported in the USDA Pest ID database have been subject to phytosanitary irradiation research (Table 4 ). There may also be a bias towards genera which are amenable to laboratory rearing or field-capture in sufficient quantities for use in quarantine research. The need for an unbiased selection of species for proper application of the SSD method reduces the importance in testing specific species of economic importance. This may prove advantageous to phytosanitary treatment researchers, as often these species require special precautions, including working under quarantine conditions, or conducting the research in their native range. The SSD approach values testing more species from a greater taxonomic diversity but is agnostic with regards to the economic importance of those species. As such, researchers can conduct studies from members of the target taxa present in their area, making the research logistically simpler and allowing for more species to be tested. Methods of measuring taxonomic representatives of a sample abound 51 , 52 and would be a valuable component for generating generic doses at higher taxonomic levels (i.e. an order level generic dose for Coleoptera). However, it is likely that National Plant Protection Organizations will still favor having testing done on the important economic species before adopting a generic dose.
The sample size of 15 species used to produce the SSD for Tephritidae is considered marginally sufficient 53 , however the maximum likelihood estimator used to fit the data to a distribution is known to exhibit sample size bias. The Cox-Snell method for small sample size bias adjustment indicated that dose estimates are likely to decline if more species are included in the analyses. As both the censored estimates and the sample-size bias corrected estimates resulted in lower recommended generic doses there is evidence that the SSD approach will result in overestimates when data quality is poor or when the number of available studies is sparse. While efforts should be made to maximize the quality of data and increase the number of species studied, for the purpose of setting regulatory requirements our results suggest the crude SSD approach may result in conservative doses when data quality/quantity is sub-optimal.
As generic doses are produced through the analysis of research from a variety of sources, collaboration and cooperation between researchers is essential if the SSD approach is to be used to set and validate new generic doses. Specifically, the data being used to generate the SSD must be of similar quality or produced and reported in such a manner that differences in data quality (i.e., number of replicates, number of doses tested) can be accounted for in the SSD model. We recommend considering the following when undertaking research to support a generic treatment:
Specify the scope of the generic dose as accurately as possible (e.g., Genus, Family or Order)
Select species for testing which represent the scope as defined previously. The number of species should be sufficient to capture the taxonomic diversity within the group. We recommend a minimum of 10 species 54 , though 13 or more is preferable 53 . Economically important species should be included, but quantity and diversity may prove more important.
Identify a uniform end point and target lethality/efficacy (i.e., an ED99.99), ideally selected under a risk-based framework.
Produce data that includes the estimated dose to achieve the targeted level of efficacy and describes the error associated with the estimate. This requires testing doses that do not provide 100% efficacy. Regression or a comparable approach is preferred.
If confirmatory testing is to be done, select the lowest dose possible where 100% treatment efficacy is expected (avoid gross overestimation).
The advancements in the ecotoxicological application of species sensitivity distributions may have parallel applications for generic doses. For example, Duboudin et al. 55 and Hiki & Iwasaki 56 investigated whether acute SSDs can predict chronic SSDs 55 , 57 . A generic dose equivalent would be to ask whether lethal endpoint SSDs can predict sterility endpoint SSDs (or vice versa). Another example is the efforts to use phylogeny and species traits to make predictions about sensitivity to various toxicants 56 , 58 , 59 , 60 . Assuming phylogenetic relationships are reliably informative of radiotolerance, then a species whose susceptibility to phytosanitary irradiation is predicted to be lower than the generic dose generated by the SSD can be treated with a lower dose, providing savings and efficiencies to treatment operators. More generally, refinements to model selection, techniques for addressing data quality concerns, and the application of Bayesian methods can be applied to improve the reliability of generic dose estimates made using species sensitivity distributions 54 , 61 , 62 , 63 .
This work shows that species sensitivity distributions can be produced from phytosanitary irradiation data, with resulting estimates and error terms that align with expectations and current approved doses. Although this technique seems particularly well suited for developing generic irradiation treatments, it may not be as suitable for other treatment types. For example, for cold treatment, cold tolerance can vary by both insect species and by commodity for the same insect species 64 , 65 , 66 and the models would need to account for multiple variables. Due to this additional factor, it may be more difficult to use SSD for developing generic cold treatments. The parametric SSD approach used in this study was derived from prior ecotoxicological work designed to determine the HC 5 for chemicals in aquatic environments, and it must be acknowledged that the generic dose has significant differences with regards to the source data and the end purpose. As such, future efforts are needed to validate and optimize the methodology for applying SSD analysis to phytosanitary purposes, with the goal ultimately being an SSD approach for phytosanitary generic dose setting which stands independent of its ecotoxicological origins.
Materials and methods
Data sources.
The reported doses used in our SSD analysis were obtained from a review of the relevant studies on phytosanitary irradiation of members of the Coleopteran family Curculionidae and the Dipteran family Tephritidae. These datasets are provided in the supplementary file , supplementary file . The studies used to generate the data set for Curculionidae were identified through a prior review of the literature conducted during an APHIS treatment approval request and are an expansion on prior reviews of phytosanitary irradiation of Curculionidae which had resulted in a recommended 150 Gy generic dose for this family 24 , 25 . Recorded doses for Curculionidae were limited to studies where the treatment endpoint was failure of F1 development at the reported dose (i.e., treated individuals may oviposit but eggs either fail to hatch or fail to develop into adults). The literature on Curculionidae irradiation included studies which used crosses of treated x untreated organisms, however for our purposes only crosses where both sexes were treated were included as this represents what is expected in a phytosanitary treatment where the entire consignment receives no less than the minimum required dose. Furthermore, when testing was conducted separately for male and female Curculionidae, we recorded the effective dose for females, as the treatment endpoint measured as failure to complete development of the F1 progeny produced by the female. Studies which focused on finding the sterilizing doses for males for the purpose of a sterile release program were deemed not suitable when the resulting level of sterility was less than 100%. This review resulted in phytosanitary radiation doses for 28 species. The lowest dose which resulted in 0 survivors, along with the number of individuals tested at that dose, was recorded for all studies which met our criteria and is presented in Table 1 . Outliers were removed from the Curculionidae dataset used in the crude analysis based on Cook’s distance as determined by a linear model specified as Reported dose ~ n + F, where n is the number of specimens treated in the study and F is the highest dose where treatment failure was observed. Observations where Cook’s distance was greater than 4 times the mean were removed. This resulted in the removal of a single observation where the dose selected (250 Gy) appeared to be arbitrarily high, with only a single dose tested 38 .
The phytosanitary irradiation treatments for Tephritidae included in our analysis were restricted to those presented in Hallman & Loaharanu 12 with the addition of data from Follett & Armstrong 13 and Zhao et al. 67 . The treatment endpoint for Tephritidae was the failure of normal adult emergence from treated late instar larvae.
When multiple effective doses were presented for a species (usually resulting from independent studies) these data were combined to provide a single dose for that species. The combined dose was determined by taking the geometric mean of the reported doses for that species, weighted using the confidence of 99.99% efficacy where confidence was calculated as 7 :
When multiple doses were reported in the literature where C was greater than 0.9999 only the lowest of these doses was retained.
Curculionidae and tephritidae crude SSD analysis
Our approach to SSD curve fitting involved fitting the reported phytosanitary doses obtained from the literature review to four distributions; the log-normal, log-logistic, gamma and Weibull, through maximum likelihood estimation using the function ‘ssd_fit_dists’ in the R package ‘ssdtools’ 68 . We refer to this analysis as the “crude” analysis as the doses obtained during the data collection phase were not adjusted outside the use of weighted averages for species with multiple reported doses. The distribution with the lowest Akaike’s information criterion (AIC) score, adjusted for sample size, was selected for further analysis 69 . The value for dose ( x ) of the fitted cumulative distribution function at p = 0.90, 0.95 and 0.99 along with 90% confidence intervals was calculated at each value of p from the resulting parametric bootstrap (5,000 iterations) of the distribution fit . This result is analogous to the HC 5 values (hazardous concentration to 5% or less of species within the target community) in ecotoxicological studies, in which p = 0.05. In keeping with the SSD literature, we refer to the GD p value as the generic dose in which taxonomic coverage is estimated to be p , expressed as either a proportion or a percent (i.e., a GD95 value implies the dose is effective for 95% of the taxon). In addition, the obtained treatment coverage, p , as a percent, when the dose was set at proposed generic doses of 150 and 175 Gy for Curculionidae 25 and 150 Gy for Tephritidae 12 was calculated using the ‘ssd_hp’ function in the R package ‘ssd_tools’.
Interval censored SSD estimates for curculionidae
The quality of the data supporting the reported doses for Curculionidae in the literature was highly variable; the sample size n ranged from 8 to over 60,000 insects, while other studies lacked testing at multiple doses or doses below the reported dose of no observed progeny. The reported doses could thus be considered censored estimates of varying interval widths where the narrower the interval the more precise the estimate of the phytosanitary dose. We created balanced censored intervals such that, for the entire dataset, the mean difference between the lower end of the censored interval and the reported dose was approximately equal to the mean difference between the upper end of the censored interval and the reported dose. To do this we set the lower interval limit as the highest dose tested which resulted in less than 100% sterility, with the minimum value being 25 Gy, equivalent to the lowest effective dose reported in the literature . We then set the upper interval U for each reported dose D by adding the product of the confidence deficit and a factor A (Eq. 2 ), where the confidence deficit is calculated as 1- C , with C being the confidence in the reported dose being 99.99% effective (Eq. 1 ). The value for A was calculated using Eq. 3 , where \({u}_{l}\) is the mean difference between the lower interval value and the reported dose D and \({u}_{c}\) is the mean confidence value \(C\) .
This approach for setting the upper interval reflects the fact that dose estimates produced from studies with large numbers of specimens tested represent the upper limit of what the true phytosanitary dose should be and that testing at sub-effective doses provides an estimate for the lower limit. The interval values were used for the SSD analysis following the same method as the “crude” analysis, with the additional specification of the intervals included when using the “ssd_fit_dists” function to determine the appropriate distribution for the SSD analysis. The values for GD90, GD95, and GD99, along with the expected coverage at 150 Gy and 175 Gy were calculated following the same method used for the “crude” analysis.
To demonstrate the influence of sample size bias on the generic dose estimates we used the Cox-Snell method for a gamma distribution (function “coxsnell.bc in the R package ‘mle.tools’) to correct for sample size bias 49,50. The resulting shape and scale parameters of the gamma distribution for the original model fit and the bias corrected fit were used to determine the dose required to achieve GD90, GD95 and GD99 coverage via the quantile function for the gamma distribution (function ‘qgamma’ in the R package ‘ssdtools’) with p set to 0.90, 0.95 and 0.99. The coverage at 150 Gy for the original model and bias corrected model were calculated using the R function ‘pgamma’ with q set to 150 Gy.
Data availability
All data generated or analyzed during this study are included in this published article and its Supplementary Information files.
Pimentel, D., Zuniga, R. & Morrison, D. Update on the environmental and economic costs associated with alien-invasive species in the United States. Ecol. Econ. https://doi.org/10.1016/j.ecolecon.2004.10.002 (2005).
Article Google Scholar
Linders, T. E. W. et al. Direct and indirect effects of invasive species: Biodiversity loss is a major mechanism by which an invasive tree affects ecosystem functioning. J. Ecol. https://doi.org/10.1111/1365-2745.13268 (2019).
Campbell, F. T. The science of risk assessment for phytosanitary regulation and the impact of changing trade regulations. Bioscience https://doi.org/10.1641/0006-3568(2001)051[0148:TSORAF]2.0.CO;2 (2001).
Paini, D. R. et al. Global threat to agriculture from invasive species. Proc. Natl. Acad. Sci. U. S. A. https://doi.org/10.1073/pnas.1602205113 (2016).
Article PubMed PubMed Central Google Scholar
Westphal, M. I., Browne, M., MacKinnon, K. & Noble, I. The link between international trade and the global distribution of invasive alien species. Biol. Invasions https://doi.org/10.1007/s10530-007-9138-5 (2008).
Hennessey, M. et al. Phytosanitary Treatments. In The Handbook of Plant Biosecurity (eds Gordh, G. & Mckirdy, S.) 269–308 (Springer, Dordrecht, 2014).
Chapter Google Scholar
Melvin Couey, H. & Chew, V. Confidence limits and sample size in quarantine research. J. Econ. Entomol. 79 , 887–890 (1986).
Schortemeyer, M. et al. Appropriateness of probit-9 in the development of quarantine treatments for timber and timber commodities. J. Econ. Entomol. 104 , 717–731 (2011).
Article CAS PubMed Google Scholar
Haack, R. A., Uzunovic, A., Hoover, K. & Cook, J. A. Seeking alternatives to probit 9 when developing treatments for wood packaging materials under ISPM No. 15. EPPO Bull. 41 , 39–45 (2011).
Liqudio, N. J., Griffin, R. L. & Vick, K. W. Quarantine security for commodities: current approaches and potential strategies. In Proceedings of Joint Workshops of the Agricultural Research Service and the Animal and Plant Health Inspection Service, June 5–9 and July 31 -August 5, 1995 56 (1997).
Follett, P. A. Phytosanitary irradiation for fresh horticultural commodities: Generic treatments, current issues, and next steps. Stewart Postharvest Rev. 3 , 1–7 (2014).
MathSciNet Google Scholar
Hallman, G. J. & Loaharanu, P. Generic ionizing radiation quarantine treatments against fruit flies (Diptera: Tephritidae) proposed. J. Econ. Entomol. 95 , 893–901 (2002).
Article PubMed Google Scholar
Follett, P. A. & Armstrong, J. W. Revised irradiation doses to control melon fly, mediterranean fruit fly, and oriental fruit fly (Diptera: Tephritidae) and a generic dose for tephritid fruit flies. J. Econ. Entomol. 97 , 1254–1262 (2004).
Follett, P. A. & Snook, K. Irradiation for quarantine control of the invasive light brown apple moth (Lepidoptera: Tortricidae) and a generic dose for tortricid eggs and larvae. J. Econ. Entomol. 105 , 1971–1978 (2013).
Hallman, G. J., Arthur, V., Blackburn, C. M. & Parker, A. G. The case for a generic phytosanitary irradiation dose of 250Gy for Lepidoptera eggs and larvae. Radiat. Phys. Chem. 89 , 70–75 (2013).
Article ADS CAS Google Scholar
Hallman, G. J. Generic phytosanitary irradiation dose of 300 Gy proposed for the Insecta excluding pupal and adult Lepidoptera . Florida Entomol. 99 , 206–210 (2016).
Google Scholar
IPPC. ISPM 28. Annex 39. Irradiation treatment for the genus Anastrepha. 1–6 (2021).
IPPC. ISPM 28. Annex 7. Irradiation Treatment for fruit flies of the family Tephritidae (generic). 1–6 (2021).
Posthuma, L., Suter, G. W. & Traas, T. P. Species sensitivity distributions in ecotoxicology. Species sensitivity distributions in ecotoxicology (CRC Press, 2002). https://doi.org/10.1201/9781420032314 .
Book Google Scholar
Newman, M. C. et al. Applying species-sensitivity distributions in ecological risk assessment: Assumptions of distribution type and sufficient numbers of species. Environ. Toxicol. Chem. 19 , 508–515 (2000).
CAS Google Scholar
van Straalen, N. M. & van Leeuwen, C. J. European history of species sensitivity distributions. In Species Sensitivity Distributions in Ecotoxicology 43–60 (CRC Press, 2001). Doi: https://doi.org/10.1201/9781420032314.ch3 .
ANZECC & ARMCANZ. Australian and New Zealand guidelines for fresh and marine water quality. aquatic ecosystems. Aust. New Zeal. Environ. Conserv. Counc. Agric. Resour. Manag. Counc. Aust. New Zeal. 1–103 (2000).
Aldenberg, T. & Jaworska, J. S. Uncertainty of the hazardous concentration and fraction affected for normal species sensitivity distributions. Ecotoxicol. Environ. Saf. 46 , 1–18 (2000).
Hallman, G. J. Generic phytosanitary irradiation treatment for “true weevils” (Coleoptera: Curculionidae) infesting fresh commodities. Florida Entomol. 99 , 197–201 (2016).
Follett, P. A. Irradiation for quarantine control of coffee berry borer, hypothenemus hampei (coleoptera: Curculionidae: Scolytinae) in coffee and a proposed generic dose for snout beetles (coleoptera: Curculionoidea). J. Econ. Entomol. 111 , 1633–1637 (2018).
Article CAS PubMed PubMed Central Google Scholar
Earle, N. W., Simmons, L. A. & Nilakhe, S. S. Laboratory studies of sterility and competitiveness of boll weevils irradiated in an atmosphere of nitrogen, carbon dioxide, or air. J. Econ. Entomol. 72 , 687–691 (1979).
Follett, P. A., McQuate, G. T., Sylva, C. D. & Swedman, A. Sensitivity of the quarantine pest rough Sweetpotato weevil , Blosyrus asellus to postharvest irradiation treatment. Proc. Hawaiian Entomol. Soc. 48 , 23–27 (2016).
Hallman, G. J. Ionizing irradiation quarantine treatment against plum curculio (Coleoptera: Curculionidae). J. Econ. Entomol. 96 , 1399–1404 (2003).
Jacklin, S. W., Richardson, E. C. & Yonce, C. E. Substerilizing doses of gamma irradiation to produce population suppression in plum curculio1. J. Econ. Entomol. 63 , 1053–1057 (1970).
Yoshida, T., Fukami, J. I., Fukunaga, K. & Matsuyama, A. Control of harmful insects in timbers by irradiation: doses required for sterilization and inhibition of emergence of the minute pine bark beetle, Cryphalus fulvus . Jpn. J. Appl. Entomol. Zool. 18 , 52–58 (1974).
Follett, P. A. Irradiation as a methyl bromide alternative for postharvest control of Omphisa anastomosalis (Lepidoptera: Pyralidae) and euscepes postfasciatus and cylas formicarius elegantulus (Coleoptera: Curculionidae) in sweet potatoes. J. Econ. Entomol. 99 , 32–37 (2006).
Gould, W. P. & Hallman, G. J. Irradiation disinfestation of diaprepes root weevil (Coleoptera: Curculionidae) and papaya fruit fly (Diptera: Tephritidae). Florida Entomol. 87 , 391–392 (2004).
van Haandel, A. et al. Tolerance of Hylurgus ligniperda (F.) (Coleoptera: Scolytinae) and Arhopalus ferus (Mulsant) (Coleoptera: Cerambycidae) to ionising radiation: a comparison with existing generic radiation phytosanitary treatments. New Zeal. J. For. Sci. 47 , 1–9 (2017).
Burgess, E. E. & Bennett, S. E. Sterilization of the male alfalfa weevil (Hypera postica: Curculionidae) by X-Radiation. J. Econ. Entomol. 59 , 268–270 (1966).
Wood, D. L. & Stark, R. W. The effects of gamma radiation on the biology and behavior of adult ips confusus (LeConte) (Coleoptera: Scolytidae). Can. Entomol. 98 , 1–10 (1966).
Wang, X. et al. Effect of X-ray (9 MeV) irradiation on the development and propagation of Ips sexdentatus. Plant Quar. 25 , 28–31 (2011).
Zhan, G. et al. Effect of irradiation on development and propagation of larch bark beetle (Coleoptera: Scolytoidea). J. Nucl. Agric. Sci. 25 , 1200–1205 (2011).
Gerstle, C. & Sazo, L. Efecto de las radiaciones de Cesio 137 sobre la fertilidad de hembras de Naupactus xanthographus (Germar) (Coleoptera: Curculionidae). Cienc. e Investig. Agrar. 16 , 69–73 (1989).
Manoto, E. C., Obra, G. B., Reyes, M. R. & Resilva, S. S. Irradiation as a quarantine treatment for ornamentals. IAEA-Tecdoc 1082 , 81–91 (1999).
Duvenhage, A. J. & Johnson, S. A. The potential of irradiation as a postharvest disinfestation treatment against phlyctinus callosus (Coleoptera: Curculionidae). J. Econ. Entomol. 107 , 154–160 (2014).
Jaynes, A. & Godwin, P. A. Sterilization of the white-pine weevil with gamma radiation. J. Econ. Entomol. 50 , 393–395 (1957).
Article CAS Google Scholar
Aldryhim, Y. N. & Adam, E. E. Efficacy of gamma irradiation against Sitophilus granarius (L.) (Coleoptera: Curculionidae). J. Stored Prod. Res. 35 , 225–232 (1999).
Follett, P. A. et al. Irradiation quarantine treatment for control of Sitophilus oryzae (Coleoptera: Curculionidae) in rice. J. Stored Prod. Res. 52 , 63–67 (2013).
Hu, T., Chen, C. C. & Peng, W. K. Lethal effect of gamma irradiation on Sitophilus zeamais (Coleoptera: Curculionidae). Formos. Entomol. 23 , 145–150 (2003).
Arthur, V. & Wiendl, F. M. Comportamento e competitividade sexual de adultos de Sphenophorus levis Vaurie, 1978 (col., Curculionidae), uma praga da cana-de-açucar, irradiados com radiações gama do cobaldo-60. Brazilian J. Agric. 68 , 57–66 (1993).
Obra, G. B., Resilva, S. S., Follett, P. A. & Lorenzana, L. R. J. Large-scale confirmatory tests of a phytosanitary irradiation treatment against Sternochetus frigidus (Coleoptera: Curculionidae) in Philippine mango. J. Econ. Entomol. 107 , 161–165 (2014).
Seo, S. T. et al. Mango weevil: Cobalt-60 γ-irradiation of packaged mangoes. J. Econ. Entomol. 67 , 504–505 (1974).
Yoshida, T., Fukami, J. I., Fukunaga, K. & Matsuyama, A. Effects of gamma radiation on Xyleborus perforans (Wollaston) pupae and adults. J. Pestic. Sci. 2 , 413–420 (1977).
Yoshida, T., Fukami, J. I., Fukunaga, K. & Matsuyama, A. Control of the harmful insects in timbers by irradiation: Doses required for kill, sterilization and inhibition of emergence in three species of ambrosia beetles (Xyleborini) in Japan. Jpn. J. Appl. Entomol. Zool. 19 , 193–202 (1975).
Follett, P. A. & McQuate, G. T. Accelerated development of quarantine treatments for insects on poor hosts. J. Econ. Entomol. https://doi.org/10.1603/0022-0493-94.5.1005 (2001).
Plazzi, F., Ferrucci, R. R. & Passamonti, M. Phylogenetic representativeness: A new method for evaluating taxon sampling in evolutionary studies. BMC Bioinform. 11 , 1–15 (2010).
Moore, D. R. J., Priest, C. D., Galic, N., Brain, R. A. & Rodney, S. I. Correcting for phylogenetic autocorrelation in species sensitivity distributions. Integr. Environ. Assess. Manag. 16 , (2020).
Carr, G. J. & Belanger, S. E. SSDs revisited: Part I—A framework for sample size guidance on species sensitivity distribution analysis. Environ. Toxicol. Chem. 38 , 1514–1525 (2019).
Wheeler, J. R., Grist, E. P. M., Leung, K. M. Y., Morritt, D. & Crane, M. Species sensitivity distributions: Data and model choice. Mar. Pollut. Bull. 45 , 192–202 (2002).
Duboudin, C., Ciffroy, P. & Magaud, H. Acute-to-chronic species sensitivity distribution extrapolation. Environ. Toxicol. Chem. 23 , 1774–1785 (2004).
Esteves, S. M. et al. Can we predict diatoms herbicide sensitivities with phylogeny? Influence of intraspecific and interspecific variability. Ecotoxicology 26 , 1065–1077 (2017).
Hiki, K. & Iwasaki, Y. Can we reasonably predict chronic species sensitivity distributions from acute species sensitivity distributions?. Environ. Sci. Technol. 54 , 13131–13136 (2020).
Article ADS CAS PubMed Google Scholar
Baird, D. J. & Van den Brink, P. J. Using biological traits to predict species sensitivity to toxic substances. Ecotoxicol. Environ. Saf. 67 , 296–301 (2007).
Guénard, G., von der Ohe, P. C., Walker, S. C., Lek, S. & Legendre, P. Using phylogenetic information and chemical properties to predict species tolerances to pesticides. Proc. R. Soc. B Biol. Sci. 281 , 1–9 (2014).
Larras, F., Keck, F., Montuelle, B., Rimet, F. & Bouchez, A. Linking diatom sensitivity to herbicides to phylogeny: A step forward for biomonitoring?. Environ. Sci. Technol. 48 , 1921–1930 (2014).
Hayashi, T. I. & Kashiwagi, N. A bayesian method for deriving species-sensitivity distributions: Selecting the best-fit tolerance distributions of taxonomic groups. Hum. Ecol. Risk Assess. 16 , 251–263 (2010).
Xu, F. L. et al. Key issues for the development and application of the species sensitivity distribution (SSD) model for ecological risk assessment. Ecol. Indic. 54 , 227–237 (2015).
Dowse, R., Tang, D., Palmer, C. G. & Kefford, B. J. Risk assessment using the species sensitivity distribution method: Data quality versus data quantity. Environ. Toxicol. Chem. 32 , 1360–1369 (2013).
Dias, V. S. et al. Relative tolerance of three morphotypes of the anastrepha fraterculus complex (Diptera: Tephritidae) to cold phytosanitary Treatment. J. Econ. Entomol. 113 , 1176–1182 (2020).
Myers, S. W., Cancio-Martinez, E., Hallman, G. J., Fontenot, E. A. & Vreysen, M. J. B. Relative tolerance of six Bactrocera (Diptera: Tephritidae) species to phytosanitary cold treatment. J. Econ. Entomol. 109 , 2341–2347 (2016).
Gazit, Y., Akiva, R. & Gavriel, S. Cold tolerance of the Mediterranean fruit fly in date and mandarin. J. Econ. Entomol. 107 , 1745–1750 (2014).
Zhao, J. et al. Gamma radiation as a phytosanitary treatment against larvae and pupae of Bactrocera dorsalis (Diptera: Tephritidae) in guava fruits. Food Control 72 , 360–366 (2017).
Thorley, J. & Schwarz, C. ssdtools: An R package to fit Species sensitivity distributions. J. Open Sour. Softw. 3 , 1–2 (2018).
Burnham, K. P. & Anderson, D. R. Model Selection and Multimodel Inference: A Practical Information-Theoritic Approach 2nd edn. (Springer, 2002). https://doi.org/10.1007/978-0-387-22456-5_7 .
Book MATH Google Scholar
Mazucheli, J., Menezes, A. F. B. & Nadarajah, S. mle.tools: An R package for maximum likelihood bias correction. R. J. 9 , 268–290 (2017).
Cox, D. R. & Snell, E. J. A general definition of residuals. J. R. Stat. Soc. Ser. B 30 , 248–265 (1968).
MathSciNet MATH Google Scholar
Follett, P. A. Irradiation as a quarantine treatment for mango seed weevil (Coleoptera: Curculionidae). Proc. Hawaii. Entomol. Soc. 35 , 95–100 (2001).
Download references
Acknowledgements
We would like to thank Dr. Rachel King, Dr. Laura Jeffers, Dr. Amrit Karki and Dr. Heike Meissner (USDA-APHIS-PPQ) for providing helpful feedback during the development of this manuscript.
Author information
Authors and affiliations.
USDA-APHIS-PPQ-S&T Treatment and Inspection Methods Laboratory, Miami, FL, USA
Cory Penca, Andrea L. Beam & Woodward D. Bailey
You can also search for this author in PubMed Google Scholar
Contributions
C.P. developed the initial concept, conducted the analysis, and prepared the manuscript. C.P., A.L.B., and W.B. contributed to interpreting the results, refining the analysis, and proofing the manuscript.
Corresponding author
Correspondence to Cory Penca .
Ethics declarations
Competing interests.
The authors declare no competing interests. This research may not necessarily express United States Department of Agriculture (USDA)’s views. Mention of trade names or commercial products in this publication is solely for the purpose of providing specific information and does not imply recommendation or endorsement by the USDA.
Additional information
Publisher's note.
Springer Nature remains neutral with regard to jurisdictional claims in published maps and institutional affiliations.
Supplementary Information
Supplementary information 1., supplementary information 2., rights and permissions.
Open Access This article is licensed under a Creative Commons Attribution 4.0 International License, which permits use, sharing, adaptation, distribution and reproduction in any medium or format, as long as you give appropriate credit to the original author(s) and the source, provide a link to the Creative Commons licence, and indicate if changes were made. The images or other third party material in this article are included in the article's Creative Commons licence, unless indicated otherwise in a credit line to the material. If material is not included in the article's Creative Commons licence and your intended use is not permitted by statutory regulation or exceeds the permitted use, you will need to obtain permission directly from the copyright holder. To view a copy of this licence, visit http://creativecommons.org/licenses/by/4.0/ .
Reprints and permissions
About this article
Cite this article.
Penca, C., Beam, A.L. & Bailey, W.D. The applicability of species sensitivity distributions to the development of generic doses for phytosanitary irradiation. Sci Rep 13 , 2358 (2023). https://doi.org/10.1038/s41598-023-29492-1
Download citation
Received : 24 June 2022
Accepted : 06 February 2023
Published : 09 February 2023
DOI : https://doi.org/10.1038/s41598-023-29492-1
Share this article
Anyone you share the following link with will be able to read this content:
Sorry, a shareable link is not currently available for this article.
Provided by the Springer Nature SharedIt content-sharing initiative
By submitting a comment you agree to abide by our Terms and Community Guidelines . If you find something abusive or that does not comply with our terms or guidelines please flag it as inappropriate.
Quick links
- Explore articles by subject
- Guide to authors
- Editorial policies
Sign up for the Nature Briefing newsletter — what matters in science, free to your inbox daily.

- Bibliography
- More Referencing guides Blog Automated transliteration Relevant bibliographies by topics
- Automated transliteration
- Relevant bibliographies by topics
- Referencing guides
Dissertations / Theses on the topic 'Bird species richness'
Create a spot-on reference in apa, mla, chicago, harvard, and other styles.
Consult the top 35 dissertations / theses for your research on the topic 'Bird species richness.'
Next to every source in the list of references, there is an 'Add to bibliography' button. Press on it, and we will generate automatically the bibliographic reference to the chosen work in the citation style you need: APA, MLA, Harvard, Chicago, Vancouver, etc.
You can also download the full text of the academic publication as pdf and read online its abstract whenever available in the metadata.
Browse dissertations / theses on a wide variety of disciplines and organise your bibliography correctly.
De, Camargo Rafael Xavier. "Effects of Habitat Change on Bird Species Richness in Ontario, Canada." Thèse, Université d'Ottawa / University of Ottawa, 2013. http://hdl.handle.net/10393/26258.
Dyer, E. "A global study of the distribution and richness of alien bird species." Thesis, University College London (University of London), 2016. http://discovery.ucl.ac.uk/1475851/.
Hernandez-Acevedo, Jose-Dagoberto. "Reconciling spatial and temporal patterns of bird species richness in North and Central America." Thesis, University of Ottawa (Canada), 2002. http://hdl.handle.net/10393/22586.
Carnicer, Cols Jofre. "Species richness, interaction networks, and diversification in bird communities: a synthetic ecological and evolutionary perspective." Doctoral thesis, Universitat Autònoma de Barcelona, 2007. http://hdl.handle.net/10803/3690.
Lessig, Heather. "Species Distribution and Richness Patterns of Bird Communities in the High Elevation Forests of Virginia." Thesis, Virginia Tech, 2008. http://hdl.handle.net/10919/35899.
Munyenyembe, F. E., and n/a. "Bird density and species richness in suburban Canberra, Australia : relationships with street vegetation, age of suburb and distance from bird source areas of native vegetation." University of Canberra. Applied Science, 1985. http://erl.canberra.edu.au./public/adt-AUC20061027.115542.
Hiding, Camilla. "Diversity of birds in relation to area, vegetation structure and connectivity in urban green areas in La Paz, Bolivia." Thesis, Linköpings universitet, Institutionen för fysik, kemi och biologi, 2012. http://urn.kb.se/resolve?urn=urn:nbn:se:liu:diva-79408.
Desrochers, Rachelle. "Habitat Loss and Avian Range Dynamics through Space and Time." Thesis, Université d'Ottawa / University of Ottawa, 2011. http://hdl.handle.net/10393/20374.
Broshot, Nancy Ellen. "The Effects of Urbanization and Human Disturbance Upon Plant Community Structure and Bird Species Richness, Diversity, and Abundance in a Natural Forested Area (Forest Park) in Portland, Oregon." PDXScholar, 1999. https://pdxscholar.library.pdx.edu/open_access_etds/3962.
Eichinski, Philip. "Smart sampling of environmental audio recordings for biodiversity monitoring." Thesis, Queensland University of Technology, 2018. https://eprints.qut.edu.au/123022/1/Philip_Eichinski_Thesis.pdf.
Walker, Kevin R. "Climatic Dependence of Terrestrial Species Assemblage Structure." Thèse, Université d'Ottawa / University of Ottawa, 2013. http://hdl.handle.net/10393/23697.
Pettersson, Anna. "Förekomst av fågelarter i skogsdominerade och åkerdominerade hagmarker i södra och centrala Sverige." Thesis, Linköpings universitet, Institutionen för fysik, kemi och biologi, 2018. http://urn.kb.se/resolve?urn=urn:nbn:se:liu:diva-148867.
Nicolakakis, Nektaria. "Innovation rate, brain size and species richness in birds." Thesis, McGill University, 2001. http://digitool.Library.McGill.CA:80/R/?func=dbin-jump-full&object_id=31280.
Stroppel, David J. Ryan Mark R. Hubbard Mike. "Evaluation of patch-burn grazing on species richness and density of grassland birds." Diss., Columbia, Mo. : University of Missouri--Columbia, 2009. http://hdl.handle.net/10355/6462.
Finnicum, Nicole E. "Patterns of Avian Species Diversity Along an Urbanization Gradient in Edinburgh, Scotland." Ohio University / OhioLINK, 2012. http://rave.ohiolink.edu/etdc/view?acc_num=ohiou1338500714.
Montero, Guillermo Leonardo Florez. "Effect of human land-use and natural coverage on taxonomic and functional richness of mammals and birds at regional scale." reponame:Repositório Institucional da UFABC, 2017.
De, Camargo Rafael Xavier. "Ecological Responses of Avian Species to Land Cover Metrics at the Landscape-Level and Across Broad Spatial Extent." Thesis, Université d'Ottawa / University of Ottawa, 2018. http://hdl.handle.net/10393/37113.
Zuloaga, Villamizar Juan Gerardo. "Species Endemism: Predicting Broad-Scale Patterns and Conservation Priorities." Thesis, Université d'Ottawa / University of Ottawa, 2018. http://hdl.handle.net/10393/37149.
Chuang, Fu-Man, and 莊馥蔓. "Bird Species Richness and Seasonal Environmental Factors in East Asia and Australia." Thesis, 2010. http://ndltd.ncl.edu.tw/handle/84129485654655172172.
Chao-Nien, Koh. "Spatial Pattern of Breeding Bird Species Richness and Assemblage Composition in Northern Taiwan." 2006. http://www.cetd.com.tw/ec/thesisdetail.aspx?etdun=U0001-2107200611071600.
Koh, Chao-Nien, and 葛兆年. "Spatial Pattern of Breeding Bird Species Richness and Assemblage Composition in Northern Taiwan." Thesis, 2006. http://ndltd.ncl.edu.tw/handle/86252799251252722303.
Huang, Ke-Yen, and 黃可言. "Bird Species Richness and Primary Productivity along an Elevational Gradient in Central Taiwan." Thesis, 2011. http://ndltd.ncl.edu.tw/handle/41086067490232243383.
Lu, Ya-Jung, and 呂亞融. "Terrestrial bird species richness in urban riparian zone: hypotheses of area, isolation, productivity, heterogeneity and disturbance." Thesis, 2014. http://ndltd.ncl.edu.tw/handle/48157306007497581691.
Parente, Tiago Ferreira. "Predicting the effects of land-use and climate change on bird species richness in the Vez watershed, Portugal." Master's thesis, 2015. https://repositorio-aberto.up.pt/handle/10216/83415.
HSU, TZU-CHIN, and 徐紫欽. "Bird Species Richness Distribution Along an Elevational Gradient in Taiwan: a Comparison of Point Count and Mist Netting." Thesis, 2017. http://ndltd.ncl.edu.tw/handle/27059514242699122735.
Parente, Tiago Ferreira. "Predicting the effects of land-use and climate change on bird species richness in the Vez watershed, Portugal." Dissertação, 2015. https://repositorio-aberto.up.pt/handle/10216/83415.
Fitterer, Jessica Laura. "Modelling British Columbia’s ecosystems and avian richness using landscape-scale indirect indicators of biodiversity." Thesis, 2012. http://hdl.handle.net/1828/4104.
Nieh, Chia-hui, and 聶嘉慧. "Species Richness Pattern of Breeding Birds." Thesis, 1999. http://ndltd.ncl.edu.tw/handle/36712272987658981874.
Džamba, Roman. "Vztah funkční a druhové diversity ptáků Jižní Afriky." Master's thesis, 2013. http://www.nusl.cz/ntk/nusl-321102.
TVARDÍKOVÁ, Kateřina. "Trophic relationships between insectivorous birds and insect in Papua New Guinea." Doctoral thesis, 2013. http://www.nusl.cz/ntk/nusl-156658.
McDonald, Laurel. "The influence of patch size, landscape composition, and edge proximity on songbird densities and species richness in the northern tall-grass prairie." 2017. http://hdl.handle.net/1993/32092.
Maas, Bea. "Birds, bats and arthropods in tropical agroforestry landscapes: Functional diversity, multitrophic interactions and crop yield." Doctoral thesis, 2013. http://hdl.handle.net/11858/00-1735-0000-0022-5E77-5.
Lawson, Adam Matthew. "Latitudinal Gradients in Climatic Niche Evolution." Thesis, 2014. http://hdl.handle.net/1807/44035.
Hernová, Jana. "Vlastnosti biotopů zodpovědné za atraktivitu vojenských cvičišť pro ptáky: případová studie z vojenského újezdu Hradiště." Master's thesis, 2020. http://www.nusl.cz/ntk/nusl-435970.
Rajmonová, Lenka. "Význam remízků a jejich ekologických vlastností pro ptáky v intenzivně obhospodařované zemědělské krajině." Master's thesis, 2019. http://www.nusl.cz/ntk/nusl-404904.
- Share full article
Advertisement
Supported by
Guest Essay
The Invasive-Species Debate Is Not Always Simple

By Margaret Renkl
Ms. Renkl is a contributing Opinion writer who covers flora, fauna, politics and culture in the American South.
Where starlings are concerned, I thought my heart was a stone.
Starlings descend in great flocks on orchards and farms, decimating crops and dining on feed meant for livestock . In the air, they can bring down airplanes . Their excrement fouls city streets and walkways. And that’s just the nuisance they cause to people. European starlings also outcompete native birds for roosts and nest holes. What is there to love about a bird whose presence causes so many problems? A bird who doesn’t even belong here?
And yet, despite my deep environmental convictions, I have somehow fallen in love with starlings.
I love the gorgeous starry plumage that emerges after they molt. I love the way they can mimic nearly anything, including an elaborate array of construction noises that they have learned in this neighborhood of unceasing construction. I especially love the way they gather in great swooping, looping wintertime flocks, turning the sky into an endless blue stage for their endlessly inventive performances.
As the discourse around nonnative plants and animals grows increasingly strident , I’ve been thinking a lot about the starling-softened stone that was once my heart.
In late March, a New York chapter of Wild Ones , a national nonprofit that advocates for native plants and natural landscapes, posted an explanation for why planting spicebush is better than planting forsythia . Like forsythia, spicebush adds a pop of yellow color to the early spring garden. Like forsythia, spicebush can create a natural screen for backyard privacy. But unlike forsythia, which is both nonnative and sterile, spicebush flowers feed pollinators in springtime. Its leaves feed spicebush-swallowtail caterpillars in summer. Its berries feed a host of songbirds in fall.
One of these plants can restore a garden to its original purpose as a biodiverse ecosystem. The other simply offers a brief display of yellow flowers.
These are incontrovertible facts. Native creatures evolved to recognize native plants as food and habitat. At a time when insect populations are plummeting (in part because of the ubiquity of nonnative plants ) and two-thirds of North American birds are at risk of extinction , there is no good reason to plant a flower that offers nothing to the wild world.
But that fact didn’t stop the pro-forsythia contingent from flying into an online rage at the very idea that someone was coming for the flower they learned to love at Granny’s knee. (I won’t link to these posts because I don’t want to add fuel to the conflagration.) In a comment on one post, someone called native-plant advocates “plant racists.”
There was nothing preachy about the Wild Ones post, which after all was aimed at native-plant enthusiasts anyway, but it’s true that environmentalists can sometimes take on a give-no-quarter tone. Sometimes they advocate for a slash-and-burn approach, using literal fire or literal poison to kill off any plant that doesn’t have the right provenance. Sometimes they call for killing introduced birds by any method that works. I can see how unpleasantly similar all this sounds to the dangerous nativist impulses in our culture.
But human beings, whatever their race, belong to the same species. Depending on how you look at it, either all human beings are native to a particular ecosystem or none of us are. Plant and animal species, on the other hand, evolved for a particular landscape — a landscape that in turn evolved to accommodate their presence. Crucial habitat is lost when introduced plants crowd out the native ones that sustain indigenous wildlife.
Despite the scolding tone that native-plant advocates can sometimes take, they are making an irrefutable point. The earth is teetering near a tipping point of no return. In the context of environmental apocalypse, there is no time — and no square inch of garden space — to waste. Every Cassandra in human history has felt this way: desperate to make others see the truth before the towers are on fire.
I am one of those Cassandras. I wrote a whole book about how we can learn to be better neighbors to the wild creatures who share our ecosystems.
But I am also learning how much more complicated this question of who belongs and who does not can sometimes be. Burmese pythons are incontestably devastating the Everglades . But starlings don’t appear to have nearly the negative impact on native cavity-nesting birds that they were long presumed to have. And as the climate changes, we are seeing that it is also changing where specific plants and animals can thrive. Through seed dispersal, introduced creatures can end up being what allows native plants to survive climate change.
Eradicating all the problematic plants that Americans have introduced into their landscapes — not to mention 85 million starlings — is just never going to happen. My husband and I have been rewilding our half-acre lot with increasing urgency for the past 29 years, with only limited success. To return this tiny ecosystem to its pristine origins without using fire or poison would require a level of backbreaking work that neither of us has the back for, and I’m not sure I would risk it anyway. To smother everything in pursuit of a pure yard would mean also smothering the spring beauties and the spring beauty mining bees.
I’m certainly not arguing that what we plant in our gardens doesn’t ultimately matter. It matters very much, and I always want to be on the side of helping rather than harming. Though I have at times been misled by an inaccurate nursery tag, I would never introduce a nonnative plant on purpose. I try to control invasive vegetation, and I will keep on trying, but there is only so much I can realistically do.
Besides, where they are welcomed, wild creatures can find a way to make use of nearly everything. Even plants that feed nobody can serve as a shelter from the cold, or as a nesting site, or as a place to hide from predators. If there’s a plant already in this yard that is doing no harm, I try not to worry about it too much.
Forsythia, for example, is not on any invasive species lists . Since I’ve been honest about my love for starlings, I’ll admit that I love our stand of forsythia, too. My mother started it from a cutting. She planted it here soon after we moved into this house. I had just survived a devastating late miscarriage, and she thought its bright color might cheer me up.
I treasure the native plants that my husband and I have lovingly added to this yard, but I belong to a species that treasures loving memories, too, and I can’t help loving that useless spray of yellow flowers at the end of every fragile gray winter. It reminds me of my beautiful mother, who wanted to save me from my own grief and thought to do it the only way she knew how: by planting flowers.
Margaret Renkl , a contributing Opinion writer, is the author of the books “ The Comfort of Crows: A Backyard Year, ” “ Graceland, at Last ” and “ Late Migrations .”
The Times is committed to publishing a diversity of letters to the editor. We’d like to hear what you think about this or any of our articles. Here are some tips . And here’s our email: [email protected] .
Follow the New York Times Opinion section on Facebook , Instagram , TikTok , WhatsApp , X and Threads .
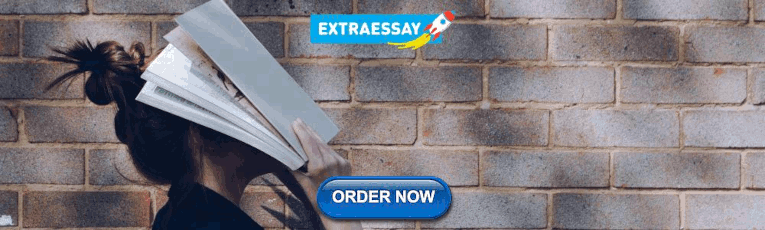
IMAGES
VIDEO
COMMENTS
invasive species, where such species are viewed as inherently "bad." Because of this negative bias, many wildlife managers, conservation biologists, and ecologists share the default view that invasive species are nuisances and must be gotten rid of, using the most cost-effective and efficient means possible.
Continental freshwaters represent among the most diverse and species-dense habitats on Earth, with almost 18,000 fish species (> 50% of all fish species) compressed into just < 0.5% of the total ...
Thesis Abstract: The reality of the modern world is that humanity is increasing its population rapidly, and demanding more resources than the earth can provide. Humans are utilizing resources like water ... many species begin their dramatic decline towards extinction and their total disappearance from this planet. Almost simultaneously with the ...
Analysis of Warren et al. ( 2018) on a global scale on the effects of climate change on the distribution of insects, vertebrates and plants indicated that even with 2 °C temperature increase, approximately 18% of insects, 16% of plants and 8% of vertebrates species are projected to loose > 50% geographic range; this falls to 6% for insects, 8% ...
Dissertations and Theses. Doctoral Dissertation Titles. Master Theses and Reports. Keller, Danielle. 2018 Ph.D. How the structure and spatial components of habitat affect estuarine communities. Payne, Christopher. 2018 Ph.D. Long-term temporal dynamics of Duke Forest.
1 Chapter 1 INTRODUCTION The studies reported in this dissertation were conducted on two species, Lygus hesperus (Knight) and Lygus lineolaris (Palisot de Beauvois), both of which are native to North America. While L. lineolaris is present throughout North America, it is the predominate Lygus species east of the Rocky Mountains and only represents a small proportion of the Lygus species complex in
Habitat loss and fragmentation has long been considered the primary cause for biodiversity loss and ecosystem degradation worldwide, and is a key research topic in landscape ecology (Wu 2013).Habitat fragmentation often refers to the reduction of continuous tracts of habitat to smaller, spatially distinct remnant patches, and habitat loss typically occurs concurrently with habitat ...
Across all species, males were more aggressive than females and Z. x-notata was the most aggressive, followed by L. sclopetarius and N. umbratica. ... Life history of the bridge spider, Larinioides sclopetarius (Clerck, 1757). Dissertation, University of Hamburg, Germany. Kleinteich A, Schneider JM (2011) Developmental strategies in an invasive ...
Pimentel et al. (2005), more than 50,000 species of plant, animal and microbes. Type of Invasive. 3 . The li terature on the e conomics of invasive species is vast. Recent journal-article length .
The rapid extinction of endangered plants (EPs) may lead to the destruction of entire ecosystems, which will seriously threaten the survival and development of humans. Research on endangered plants should be strengthened to scientifically guide the protection of endangered plants. Based on 1635 publications collected from the Web of Science Core Collection™ (WoS), this paper aims to provide ...
There was supportive evidence that, for two of the four species, the distribution of habitat influences settlement patterns of birds and thus shapes dispersal behavior in human-dominated landscapes. Finally, we used mark-recapture data to assess variation in adult survival across the rural-to-urban gradient to test hypotheses about the ...
Invasive Alien Species in a Changing World: a Juridical Analysis of a Global Challenge A Study of the Interconnection between Climate Change and Invasive Alien Species in Marine Ecosystems — Nahikari Ajubita Rubio LLM Law of the Sea, 2019 - JUR 3910 Supervisor: Vito De Lucia
Invasive species are one of the leading causes of biodiversity loss and ecosystem degradation, with considerable impacts on native species via predation and competition (Clavero and García ...
Background Ecological research now deals increasingly with the effects of noise pollution on biodiversity. Indeed, many studies have shown the impacts of anthropogenic noise and concluded that it is potentially a threat to the persistence of many species. The present work is a systematic map of the evidence of the impacts of all anthropogenic noises (industrial, urban, transportation, etc.) on ...
Thesis Utah State University;1-8. 4. Eeva, B.E., Gilyazov, A.S,, Kozlov, M.V. (2012). Pollution ... Species of birds common to both these regions were chosen and their number was recorded. The ...
The introduction of invasive species through global trade represents one of the most significant threats to global biodiversity and ecosystem services and is responsible for billions of dollars in ...
Journal article. Video (online) Consult the top 50 dissertations / theses for your research on the topic 'Fish species.'. Next to every source in the list of references, there is an 'Add to bibliography' button. Press on it, and we will generate automatically the bibliographic reference to the chosen work in the citation style you need: APA ...
Create a spot-on reference in APA, MLA, Chicago, Harvard, and other styles. Consult the top 50 dissertations / theses for your research on the topic 'Species biogeography.'. Next to every source in the list of references, there is an 'Add to bibliography' button. Press on it, and we will generate automatically the bibliographic reference to the ...
Insufficient species monitoring data could in turn contribute to misinterpretation and mismanagement, resulting in further biodiversity loss (Ferreira et al., 2016). ... We focused mainly on scientific literature, including scientific papers, dissertations and project reports. However, WDD were frequently used for conservation or management ...
resident birds (Sherpa2000). Forest is the most significant habitat for birds by supporting around 75% of all bird species. while only 45% of all bird species have adap ted to humans modified ...
Consult the top 35 dissertations / theses for your research on the topic 'Bird species richness.'. Next to every source in the list of references, there is an 'Add to bibliography' button. Press on it, and we will generate automatically the bibliographic reference to the chosen work in the citation style you need: APA, MLA, Harvard, Chicago ...
The species sensitivity distribution (SSD) is a statistical approach that is used to estimate either the concentration of a chemical that is hazardous to no more than x% of all species (the HCx) or the proportion of species potentially affected by a given concentration of a chemical.Despite a significant body of published research and critical reviews over the past 20 yr aimed at improving the ...
PhD Dissertation Department of Environmental Science and Policy College of Science George Mason University Candidate: Meadhbh Molloy Defense Date and Time: April 16, 2024 at 1:00pm Defense Location: Hybrid: Johnson Center Meeting Room D and virtually on Zoom Title: Microbiomes and Threatened Species Management: Applications for the Conservation of the Tasmanian Devil
bird species feral pigeons and their relationship with humans" a dissertation thesis submitted to b. r. d. school of biosciences sardar patel university vallabh vidyanagar gujarat, india for the partial fulfillment for degree of master of science in zoology submitted by umang sutariya examination no.: 23 july 2020 under the guidance of prof.
The Invasive Species Debate Is Not Always Simple. April 8, 2024, 5:04 a.m. ET. Gregory Halpern/Magnum Photos. Share full article. By Margaret Renkl. Ms. Renkl is a contributing Opinion writer who ...