
An official website of the United States government
The .gov means it’s official. Federal government websites often end in .gov or .mil. Before sharing sensitive information, make sure you’re on a federal government site.
The site is secure. The https:// ensures that you are connecting to the official website and that any information you provide is encrypted and transmitted securely.
- Publications
- Account settings
Preview improvements coming to the PMC website in October 2024. Learn More or Try it out now .
- Advanced Search
- Journal List
- Genes (Basel)

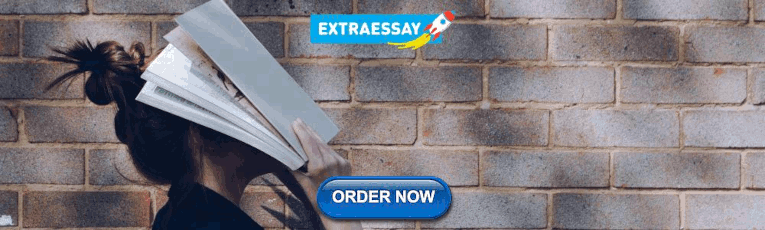
Major Depressive Disorder: Existing Hypotheses about Pathophysiological Mechanisms and New Genetic Findings
Muhammad kamran.
1 Department of Pharmacy, Faculty of Biological Sciences, Quaid-i-Azam University, Islamabad 45320, Pakistan; moc.liamg@idifimak (M.K.); kp.ude.uaq@namhera (A.u.R.)
2 Centre for Neuroimaging, Cognition and Genomics (NICOG), Discipline of Biochemistry, National University of Ireland Galway, H91 CF50 Galway, Ireland
Farhana Bibi
3 Department of Microbiology, Faculty of Biological Sciences, Quaid-i-Azam University, Islamabad 45320, Pakistan; moc.oohay@432kilamanahraf
Asim. ur. Rehman
Derek w. morris, associated data.
Data were directly downloaded from published studies and all additional generated data is contained within this manuscript.
Major depressive disorder (MDD) is a common mental disorder generally characterized by symptoms associated with mood, pleasure and effectiveness in daily life activities. MDD is ranked as a major contributor to worldwide disability. The complex pathogenesis of MDD is not yet understood, and this is a major cause of failure to develop new therapies and MDD recurrence. Here we summarize the literature on existing hypotheses about the pathophysiological mechanisms of MDD. We describe the different approaches undertaken to understand the molecular mechanism of MDD using genetic data. Hundreds of loci have now been identified by large genome-wide association studies (GWAS). We describe these studies and how they have provided information on the biological processes, cell types, tissues and druggable targets that are enriched for MDD risk genes. We detail our understanding of the genetic correlations and causal relationships between MDD and many psychiatric and non-psychiatric disorders and traits. We highlight the challenges associated with genetic studies, including the complexity of MDD genetics in diverse populations and the need for a study of rare variants and new studies of gene-environment interactions.
1. Introduction
Major depressive disorder (MDD) is a complex heterogeneous disorder and the most common psychiatric disorder [ 1 ]. It is ranked as a major contributor to worldwide disability, affecting over 300 million people, with an estimated annual prevalence of 4.4% of the total world population [ 2 ]. The World Mental Health Survey estimates a lifetime prevalence of 10–15% for MDD [ 3 ]. MDD can be chronic or recurrent in nature and is typically associated with prolonged periods of low mood and anhedonia [ 4 ]. It has significant socioeconomic consequences, including increased morbidity, disability, excess mortality, substantial economic costs and heightened risk of suicide [ 5 ]. MDD has a negative effect on an individual’s feelings, thinking and actions, causing an individual to feel sad and/or lose interest in activities that were previously enjoyable. MDD can lead to a variety of problems related to emotional and physical well-being that negatively affect an individual’s normal functioning both at work and at home [ 6 ]. In addition to characteristic mood symptoms and deviant thoughts, there are other symptoms related to cognition (mental ability to acquire knowledge through thought, experience and senses) and bodily functions (chronic fatigue, decreased interest in sex, decreased appetite, insomnia, lack of deep sleep or oversleeping). MDD is associated with many comorbid mental and physical illnesses that suggest complex underlying mechanisms. The underlying molecular mechanism(s) of MDD are still unknown.
2. Diagnosis and MDD Phenotypes
To date, there are no specific biomarkers that can be used to confirm the diagnosis of MDD for clinical or research purposes. A multifaceted approach is undertaken when diagnosing MDD that centres on a psychiatric evaluation using the Diagnostic and Statistical Manual of Mental Disorders (DSM-5), a set of diagnostic criteria developed and published by the American Psychiatric Association [ 7 ], or diagnostic criteria set out by the International Classification of Disease (ICD-10). In order to confirm the diagnosis of MDD, a physician may do a physical examination and ask questions about a patient’s health, as there can be underlying medical conditions that lead to MDD [ 8 ]. In the psychiatric evaluation, the patient is asked to fill out a questionnaire answering specific questions about symptoms, thoughts, feelings and behavior patterns [ 9 ]. DSM-5 defines a case of MDD as one where a person experiences a low mood or loss of interest or pleasure in earlier enjoyable daily activities for a period of at least two weeks, and the presence of a majority of specified symptoms such as disturbance in sleep pattern, eating, liveliness, attentiveness, or self-esteem. No omissions are specified for major depressive episode symptoms due to medical ailments, substance use disorders or medications [ 10 ]. Psychosis may also accompany MDD of varying severity [ 11 ].
No clear-cut distinction has been drawn to differentiate the depression phenotypes (probable depression, broad depression, recurrent depression, and psychotic depression). Depressive symptoms experienced may vary from individual to individual. The DSM-5 and ICD-10 classify depression based on the parameters experienced by the patient along the course of illness while considering the number of symptoms, the types of symptoms, the intensity of the symptoms and social, functional and occupational impairments in patients [ 12 ]. However, for research purposes, for example, for genetic studies [ 4 , 13 , 14 ], a range of methods has been reported to identify cases of depression in different cohorts. These methods of diagnosis range from self-reporting of depressive symptoms in biobanks, accessing electronic health records (EHRs) of individuals or scheduling structured interviews with individuals. As detailed later in Section 7 , the genetic basis of these different phenotypic definitions is highly correlated with each other.
3. Available Treatment Options for MDD
Although MDD is a treatable disorder, treatments work for some individuals and not others. Various pharmacological and non-pharmacological interventions, including psychotherapy, electroconvulsive therapy (ECT) and transcranial management stimulation, are used in the management of MDD [ 15 , 16 , 17 ]. Psychotherapy (cognitive behavior therapy, interpersonal therapy and online psychological interventions) has been reported to have an impact on relieving symptoms [ 18 ] and on improving patients’ overall quality of life [ 19 ]. Therefore, many guidelines developed for the management of MDD recommend psychotherapy as a monotherapy or in combination with antidepressant medication.
The most common antidepressants approved by the US FDA for use in the management of MDD include selective serotonin reuptake inhibitors (SSRIs), serotonin-norepinephrine reuptake inhibitors (SNRIs), monoamine oxidase inhibitors (MAOIs), tricyclic antidepressants (TCAs), noradrenergic and specific serotonergic modulators, norepinephrine-dopamine reuptake inhibitors, multimodal antidepressants, serotonin modulators, MT1/MT2 agonists and 5-HT2C antagonists, serotonin reuptake inhibitor and 5-HT1A-receptor partial agonists, neurosteroids, and newer agents like non-competitive N-methyl-D-aspartate receptor antagonists ( Table 1 ) [ 15 , 16 , 20 ]. The focus of research has been to optimize the treatment strategy for MDD. Although not used for diagnosis, individual biomarkers and clinical characteristics are used to predict the efficiency of management strategies. These strategies include the use of genetics, electrophysiology, neuroimaging, peripheral protein expression levels, neurocognitive performance, data on developmental trauma and the personality of an individual [ 21 ].
US FDA-approved antidepressants used in the management of major depressive disorder (MDD).
4. Pathophysiological Mechanisms of MDD
The characteristic symptoms of MDD that cause complexity in the treatment of the disorder lie within the domain of cognitive, emotional and physiological effects [ 20 ]. The currently available treatments, including antidepressants, are only effective for 50% of MDD patients, which reflects the fact that the diagnosis of MDD is solely dependent on behavioral symptoms. The treatment options of MDD are, therefore, not specific to the pathology of the disease [ 22 ]. Despite an abundance of research in understanding the neurophysiology and neuropsychiatry of MDD, the precise mechanism for the development of depression is still unknown due to the complex heterogeneous nature of the disorder, possibly involving multiple etiologies. It is crucial to understand the pathophysiological mechanisms of MDD to develop new therapeutic options [ 23 ].
Previous studies support the involvement of various pathophysiological mechanisms, such as the biogenic amine hypothesis, the receptor hypothesis, neurotrophic factors, cytokine theory and endocrine factors. A major challenge is that no single hypothesis explains all aspects of MDD due to the involvement of multiple interlinked disease mechanisms [ 24 , 25 , 26 ].
4.1. Biogenic Amine Hypothesis
The brain contains a vast number of serotonergic, dopaminergic and noradrenergic neurons. The process of memory working, behavioral regulation and mindfulness are all associated with the function of the prefrontal cortex, which is regulated by norepinephrine through noradrenergic neurons. Dopamine is linked with the modulation of reward and motivation, memory working and mindfulness, whereas serotonin is the largest cohesive system of neurotransmitters and innervates all areas of the brain [ 27 ]. It is well supported that several behavioral symptoms (fatigue, low mood, psychomotor retardation, reduced motivation and vigilance) are associated with monoaminergic systems. Several studies reported considerably lower levels of serotonin in depressed patient brain samples from suicide cases. Abnormalities in dopamine levels in the brain cause impaired motivation, aggregation and concentration, whereas reduced levels of norepinephrine (individually or coupled with dopamine) mediate the vast number of depressive symptoms such as aggression, sex, concentration, appetite, mindfulness and motivation [ 28 , 29 ]. The low availability of these neurotransmitters results in impaired cognitive functions and reduced neurotransmission, which consequently causes depression. Another underlying cause of depression may involve the reduced functioning of protein transporters or neurotransmitter receptors [ 30 ]. Various studies reported lower levels of monoamine neurotransmitters due to reduced numbers of transport proteins at cellular junctions in depression patients [ 31 , 32 ].
4.2. Receptor Hypothesis
The receptor hypothesis attempts to elucidate depression-related pathogenesis from multiple perspectives. There are a number of receptors, such as α-amino-3-hydroxy-5-methyl-4-isoxazolepropioni acid (AMPA), γ-aminobutyric acid (GABA), N-methyl-D-aspartate (NMDA), 5-hydroxytryptamine (5-HT), glucocorticoid and dopamine receptors, which have been extensively studied for their role in the occurrence and development of depression [ 25 ].
The AMPA receptors (heterotetrameric ion channels) are subtypes of glutamate receptors with an assemblage of four subunits (GluA1, GluA2, GluA3 & GluA4). A total of 80% of AMPA receptors are composed of the subunits GluA1 and GluA2, and the remaining 20% are composed of GluA3 and GluA4. These are found in postsynaptic membranes in the hippocampal neurons [ 33 ]. The GRIA family of genes that encodes these receptors lies in the 5q33 chromosome region. The onset of depression (aging relevant) is reported to be associated with SNPs in GRIA (rs4403097 & rs4302506). Various studies have shown changes in the distribution of AMPA subunits with MDD and an associated deterioration of plasticity related to the expression of AMPA receptors has suggested the need for the formulation of antidepressants which could potentially activate the AMPA receptors [ 34 , 35 ].
The GABA receptors are ion channels composed of four subunits (α, β, γ, δ) and are responsible for the transmission of an inhibitory neurotransmitter (GABA). These receptors are important targets of various drugs, including barbiturates, benzodiazepines and anesthetics. The gene Gabra2, which encodes the GABAA receptors (composed of 2 α1, 2 β2 and 1 γ2 subunits), is located on chromosome 4 [ 36 ]. Studies support the presence of defects in GABAergic synaptic transmissions, impaired homeostasis in the hippocampal region, and the dysfunction of glutamate-related synapses in mice samples upon GABAergic deficiency due to inhibition of genes encoding γ2 subunits, consequently resulting in depression [ 37 ].
The NMDA receptors are hetero-multimeric complexes composed of two GluN1 and two GluN2 subunits. These receptors are subtypes of ionic glutamate receptors generally expressed in the central nervous system, often associated with mood regulation. In MDD patients, the antagonists of NMDA receptors have shown positive therapeutic efficacy along with new synaptic connectivity and proficiency in reversing the neuronal deficiency induced by stress [ 38 ]. Studies have shown the abnormal expression of GluN2A (encoded by the GRIN2A gene in 16p 13.2) due to GRIN2A gene hyper-methylation, which resulted in increased susceptibility to depression [ 39 ]. Another study suggests the increased synthesis of synaptic proteins and relief in behavioral symptoms of depression upon knock out of the GRIN2B gene (located on 12p13.1), which encodes the GluN2B subunit [ 40 ]. A GWAS study has suggested that GRIN2B (based on SNP rs220549) is a potential candidate gene that is linked with the onset of depression in MDD patients [ 41 ].
Notably, 5-HT (serotonin) is an inhibitory neurotransmitter with 14 receptor subtypes spread widely in synapses and the cerebral cortex. It has been found to be one of the key factors in the pathogenesis of MDD and mediates the regulation and efficacy of antidepressant drugs [ 42 ]. A significant association was observed between the serotonin transporter gene SLC6A4 (genetic variants: 5-HTTLPR, rs140700, rs4251417, rs6354, rs25528, rs25531) and DNA methylation at various CpG sites, which suggests DNA methylation-associated depression status in a community-based population study of older individuals. Lower methylation was associated with depression in individuals homozygous for the short 5-HTTLPR and 5HTTLPR/rs25531 alleles, but only for individuals with the SS genotype of 5-HTTLPR [ 43 ]. The effect of environmental stress on gene expression and neuronal functioning through epigenetic alterations may generally represent a mechanism for disease risk. Epigenetic alterations such as changes in DNA methylation have been observed in MDD patients [ 44 ]. DNA methylation is the most-investigated epigenetic alteration in MDD patients. Generally, DNA methylation at the binding sites of enhancers results in transcriptional repression, whereas there is an antagonistic effect for DNA methylation at binding sites for repressors. Structural variations within a genomic region might also contribute to the development of the disease phenotype. For the complex structure of the SLC6A4 gene, studies have shown that the expression of this gene is controlled by the modulation of microRNA mir-16 binding sites located in non-translating regions. miR-16 was found to be a regulator (post-transcriptional) of 5-HT levels, and expression of the 5-HT gene was modulated by 3′-UTR microRNAs. It was observed that the reduction in the expression level of 5-HT upon overexpressing miR-16 changes the [125I]-RTI-55 and [3H]-paroxetine binding sites in the differential serotonergic cell line. An increased level of 5-HT expression upon miR-16 reduction was also observed. Therefore, the expression of SLC6A4 and the resultant function of 5-HTT may be strongly affected by the polymorphism within or near regions of mir-16 binding sites [ 45 ]. The first studied large-scale meta-analysis of research on depression suggested the statistically significant association of SLC6A4 and SLC6A3 genes among various genes of the monoaminergic system [ 46 ].
Glucocorticoids represent the secretion of stress-related hormones with two different receptors (low-affinity mineralocorticoid receptor (MR) and high-affinity glucocorticoid receptor (GR)) [ 47 ]. GR regulates the expression of neurotrophins that induce apoptosis in neuronal cells and alter the neurogenesis in the hippocampal region in adults [ 48 ]. The imbalance of MR and central GR causes HPA axis disorders and increased susceptibility to depression. Studies have shown affected levels of GR and HPA axis functions due to varying expression of the NR3CI gene that encodes GR (located on chromosome 5). Low levels of NR3CI expression were also found in MDD patients with suicidal attempts [ 49 ]. Epigenetic alterations have been observed in the promoter region of NR3C1 at overlapping CpG sites in peripheral blood cells and brain tissue of patients who experienced early life trauma [ 50 ].
The dopamine receptors are members of the transmembrane domain G-protein-coupled receptors family, which are involved in the transmission of noradrenaline precursor (dopamine) [ 51 ]. The dopamine (D2) receptors are a key target site for antidepressant drugs, including haloperidol [ 52 ]. A polymorphism (A241G) in the gene ( DRD2 ) encoding the D2 receptor, located on 5p 15.3, was observed, which has shown a correlation with early to mid-adolescence as well as maternal parenting-related depressive symptoms [ 53 ].
4.3. Endocrine System
Abnormalities related to the endocrine system such as alterations in growth hormone levels (GH), HPA dysfunction and abnormalities in thyroid levels have been identified as key contributors to MDD etiology [ 28 ]. The direct and indirect effects of GH on the NE system have been investigated for their contribution to the development of depression. Defective GH release was observed in depressed patients [ 54 ]. Specifically, the use of clonidine and apomorphine exhibited a blunt response of GH secretion in MDD patients [ 55 ]. The thyroid gland is responsible for producing two active forms of hormone (tri-iodothyronine T3 and tetra-iodothyronine T4) by the activation of thyroid-stimulating hormone (TSH) in the pituitary [ 56 ]. Several studies support the possible association between thyroid function alteration and depression. Thyroid hormones regulate the whole metabolic reaction in the human body. Abnormalities in thyroid functioning could possibly cause symptoms such as sleep disturbance, loss in weight, and psychomotor retardation in MDD patients [ 57 ]. Studies also suggest the indirect role of thyroid hormones as co-transmitters in the adrenergic nervous system [ 58 ].
The HPA axis is considered a significant contributor to the development of MDD in patients. In depressed patients, dysfunction in the glucocorticoid mechanism, hyper-secretion of cortisol and corticotrophin-releasing factor (CRF), the administration of exogenous glucocorticoid, reduced HPA axis suppression and impaired signaling of corticosteroid receptors have been suggested in association with alterations in the HPA axis [ 59 ]. The HPA activity is highly regulated by CRF, which increases the secretion of adrenocorticotrophic hormone (ACTH) from the interior pituitary following the stimulation of adrenal glands to secrete cortisol [ 60 ]. Several studies have reported elevated levels of CRF in the cerebrospinal fluid of depressed patients. Elevated and persistent levels of CRF are responsible for high cortisol secretion and are associated with intense and severe symptoms such as hopelessness, weight loss, disturbed sleep, psychomotor retardation and overreaction towards psychological stressors in MDD patients [ 59 , 61 ].
4.4. The Cytokine Theory
Cytokines are inflammatory chemicals secreted in response to foreign pathogenic antigens by lymphoid cells [ 62 ]. The inflammatory process in the human body, such as the regulation of monocytes, basophils, lymphocytes, neutrophils, and eosinophils, is a function of cytokines [ 63 ]. Several types of cytokines involve interleukins, C-reactive proteins, interferons, tumor necrosis factor and serum amyloid proteins [ 64 ]. The altered immune functions in MDD patients potentially indicate the role of cytokines in the development of chronic stress levels in patients. There are several studies reported in support of and in contradiction to the association of cytokines with MDD development [ 65 ]. Numerous studies have linked the inflammatory factors as a result of sickness to behavior in depression. The normal immunologic response in the human body towards pro-inflammatory cytokines and infections causes various behavioral responses, such as disturbed sleep, nausea, loss of interest and appetite, and increased body temperature, which suggest that the actions of inflammatory cytokines cause broader range of behavioral sickness responses in MDD patients [ 66 , 67 ].
4.5. Neurotrophic Factor Hypothesis
This hypothesis involves both stress hormone signaling involving cortisol and neurotrophic factor signaling, including brain-derived neurotrophic factor (BDNF) [ 68 ]. Over time, inflammatory factors in the brain and the over-excitation or over-stimulation of neuronal activity leads to a decrease in neurogenesis (formation of new neurons in the brain), contributing to the pathophysiology of MDD. Therefore, whenever there is a stressor, the brain responds to it normally by releasing cortisol [ 69 ]. The circulating levels of cortisol become higher. In a situation where the brain is unable to control or suppress the release of cortisol, it results in decreased neurogenesis. This hypothesis is relevant because most antidepressant drugs either prevent or reverse the damage caused by increased cortisol release, over-excitation, and inflammation [ 70 , 71 ]. Additionally, ECT is one possible adjunctive therapy for depression that canprevent or reverse the effects of cortisol and overexcitation [ 72 ]. The factor linking cortisol and stress to decreased neurogenesis is thought to be BDNF. BDNF normally stimulates dendritic sprouting and new neuron growth, but following the long-term elevation of cortisol, BDNF is decreased. Antidepressants are thought to reverse this effect by increasing BDNF levels [ 73 ]. In addition to the HPA axis [ 74 ] and the release of cortisol, additional neuroendocrine factors are at play in this hypothesis, such as steroids and endogenous steroids like the progesterone derivative allopregnanolone. The reason for the inclusion of allopregnanolone in this hypothesis is that chronic stress and depression are known to be associated with decreased allopregnanolone [ 75 ]. There is also a rapid decrease in this hormone following labor and delivery, which could contribute to the development of postpartum depression specifically [ 76 ]. Thyroid hormone is another neuroendocrine factor that can contribute to the expression of depression. The main evidence for this is that hypothyroidism or reduced thyroid hormone is a cause of secondary depression [ 77 ].
4.6. Neuroplasticity/Neurogenesis Hypothesis
In adults, neurogenesis is the process of the formation of new neurons and neuronal connections in a certain region of the brain (dentate gyrus of the hippocampus and lateral ventricles) [ 78 ]. According to several studies, one of the causes that describe the pathophysiology of depression is the lack of neurogenesis in adults. The disturbance in metabolic levels of neurotropic factors such as BDNF in nervous tissue is found to be the primary cause of these effects [ 24 ]. In addition, various studies have reported changes in morphological and synaptic plasticity in MDD patients [ 79 ]. In the limbic structures of adult brains, BDNF is a highly expressed neurotropic factor that maintains the survival, migration, proliferation and differentiation of neurons. The dysregulation of BDNF expression, such as an increase in DNA methylation of BDNF gene promoters, was observed in peripheral mononuclear cells of depressed patients’ blood [ 80 ]. Various studies have reported decreased levels of receptor TrkB (neurotropic receptor tyrosine kinase 2) and its genetic variant NTRK2 in depressed and suicidal patients [ 81 ]. The antidepressant drugs work to increase BDNF levels, but the exact mechanism of how they activate BDNF expression is still not so clear. A recent study has shown the probable contribution of BDNF rs2049046 and rs11030094 to increase the response of antidepressant treatment in MDD patients [ 82 ].
5. Major Risk Factors of MDD
5.1. environmental factors.
There are many factors that are likely responsible for the development of MDD, and of all these, life experiences are most studied. Risk factors related to life events and changes include but are not limited to natural calamities, financial constraints, bullying, social seclusion, social stress, diagnosis of a serious medical disorder, loss of loved ones, and childbirth [ 15 ]. The prevailing COVID-19 pandemic and the resultant restrictions have greatly affected mental health. A study conducted by Evans et al. (2021) reported a significant rise in the symptoms of MDD during the COVID-19 pandemic. Alcohol use may be a contributory factor to this [ 83 ]. The use of certain medications such as alpha-interferon, isotretinoin, and rimonabant also resulted in a higher risk of developing MDD [ 84 , 85 , 86 ].
5.2. Genetic Factors and Heritability
MDD is a multifactorial disorder caused not only by the environment but by genes as well, and this is supported by genetic and twin studies [ 87 ]. Heritability is a key concept in genetics that estimates how much of the variance in a trait is due to genetic factors, or, put another way, how much resemblance exists between parents and offspring. Heritability is typically estimated from twin studies based on the expected genetics and environmental sharing within the twin pairs. Heritability is population and time-specific [ 88 ]. The heritability of psychiatric disorders ranges from moderately to highly heritable, but the degree to which genetic variation is exclusive to individual disorders or shared among them is unclear. Twin studies and other similar studies suggest a moderately heritable component (37%) for MDD, but it may range between 26% and 49% [ 89 ]. Overall, heritability estimates from twin studies are fairly similar, but twin studies rely on some key assumptions, particularly the common environment assumptions between monozygotic and dizygotic twin pairs. This was relevant to the missing heritability reported by early genome-wide association studies (GWAS), which led some studies to suggest that the disease’s twin heritability might have been overestimated [ 90 ]. However, there is a growing literature on heritability estimation based on population-based registers or electronic health record (EHR) data. These studies reconstruct the extended pedigree based on either recorded or inferred familial relationships, and the estimates here are fairly similar to those from twin studies [ 91 , 92 , 93 ]. Heritability is significantly higher in females than in males. Most, but not all, genetic risk is shared across sexes. Given the moderate heritability of MDD, it would be useful to identify clinical subtypes that are more heritable. One successful example was the ascertainment of recurrent MDD cases in women to enhance the GWAS power [ 94 ]. Early evidence established recurrent MDD as a more heritable form of the disorder, but recent studies using larger population-based data were able to extend the evidence to suggest that disorder subtypes based on the early age of onset, comorbid anxiety disorder, higher severity of MDD and postpartum depression are more heritable forms of MDD [ 95 ].
The combination of high prevalence and low heritability has made it challenging to map genes for MDD. There has also been a debate about the genetic architecture of MDD. The common-disease/common-variant model posits that psychiatric disorders are caused by the combined effect of many common variants, each of small effect. The multiple rare variant model argues that psychiatric disorders are caused by large-effect mutations in single genes, with each case having different casual mutations. The results from genetic studies described later indicate that both models are relevant to a complex polygenic disorder such as MDD, where contributory genetic variants exist along the continua of allele frequency (from rare to common) and effect size (from small to large), with the exception of common large-effect genetic variants due to the pressures of selection. This genetic complexity requires a range of study designs to find the susceptibility genes for MDD.
6. Poor Replication of MDD-Associated Genes Identified by Candidate Gene Studies
Early research on the identification of genes for MDD focused mainly on particular candidate polymorphisms in genes hypothesized to cause depression [ 24 ]. These candidate genes were selected on the basis of their role in disordered physiological processes and drug mechanisms related to the known biological basis of the disorder [ 79 ]. More than 1500 studies have been reported that studied variants in more than 200 candidate genes with conflicting results. Studies were largely underpowered with insufficient sample sizes to detect associations with variants of low effect, resulting in inconsistent findings and failure to achieve independent replications. This intensified the need for alternative methods to the candidate gene approach for reliable, replicable genetic findings [ 95 ]. In fact, a high-level National Institute of Mental Health genomics panel recommended, “Candidate gene studies of psychopathologic, cognitive or behavioral phenotypes should be abandoned in favor of well-powered, unbiased association studies” [ 96 ]. Therefore, the candidate gene approach has been replaced with more robust GWAS that leverages large sample sizes, advanced genomics technologies such as genotyping arrays and next-generation sequencing (NGS), imputation and reference panels to provide genome-wide coverage of common SNPs and a range of biostatistical methods to detect novel and replicable genetic associations for MDD.
7. Contribution of GWAS to Our Understanding of the Genetic Architecture of MDD
GWAS test for differences in the allelic frequency of genetic variants between groups of individuals who share similar ancestry but are different phenotypically (e.g., MDD cases vs. controls). Single nucleotide polymorphisms (SNPs) are the most widely studied genetic variants in GWAS using genotyping arrays, although these arrays can also detect large copy number variants (CNVs) [ 96 , 97 ]. GWAS identify SNPs that have statistically significant associations with phenotypes of interest based on the association reaching genome-wide significance (based on a correction for all SNPs tested) and showing evidence of independent replication. Over the last 15 years, GWAS have led to some remarkable discoveries in the field of human genetics. They have successfully been applied to understand disease biology, estimate heritabilities, calculate genetic correlations between phenotypes, develop risk prediction using genetic variables, appraise drug development programmes, and draw inferences about the potential causal relationship between risk factors and health outcomes [ 98 ]. In psychiatry, the findings of GWAS for MDD and associated traits have proven to be more productive than earlier linkage studies and candidate gene association studies [ 96 ]. Hundreds of loci have now been reported in different large-sample GWAS that are significantly and strongly associated with MDD and associated traits [ 95 , 99 ].
Early GWAS by the initial Psychiatric Genomics Consortium (PGC) MDD mega-analysis (9240 cases; [ 100 ]) or by the CHARGE meta-analysis of depressive symptoms ( n = 34,549; [ 101 ]) struggled to detect any significant results. However, as sample sizes increased, numbers of genome-wide significant loci began to increase. The estimates of the proportion of variance attributable to common SNPs (SNP heritability, h 2 SNP ) remained relatively constant even after sample sizes with >100,000 cases were used ( Figure 1 ). A meta-analysis of depressive symptoms identified 2 loci (161,460 individuals; [ 17 ]), and an analysis of self-reported major depression identified 15 loci in a sample containing 75,607 cases and 231,747 controls [ 102 ]. Studies since then have identified 14 loci (113,769 cases and controls 208,811 [ 103 ]), 44 loci (135,458 cases and 344,901 controls; [ 5 ]), 102 loci (246,363 cases and 561,190 controls; [ 4 ]) and 178 loci (366,434 cases and 847,433; [ 99 ]) with continually increasing sample sizes.

A plot of the number of genome-wide significant loci (left-hand-side x -axis and blue line) and h 2 SNP (right-hand-side x -axis and orange line) from GWAS of MDD showing increases in the number of significant findings with increasing the sample sizes. From left to right, the dots represent the following studies: PGC (2013) [ 100 ], CONVERGE Consortium (2015) [ 94 ], Hyde et al. (2016) [ 102 ], Howard et al. (2018) [ 103 ], Wray et al. (2018) [ 104 ], Howard et al. (2019) [ 4 ] and Levey at al. (2021) [ 99 ].
The largest study identifying 178 loci was a bi-ancestral meta-analysis using individuals of European ancestry and African ancestry from the Million Veteran Program (MVP), 23andMe, UK Biobank and FinnGen and included many samples used in previous MDD GWAS [ 99 ]. Similar to those GWAS, this study used different depression phenotypes to maximize the case sample available for analysis. For MVP, different diagnostic definitions were available: an ICD–based phenotype using EHR data, self-reported physician diagnosis of depression and the two-item Patient Health Questionnaire scale of depressive symptoms in the past 2 weeks, included in the MVP baseline survey. Genetic correlations among these traits were high. Of these, the EHR-derived ICD codes for MDD were chosen for the primary meta-analysis because they had the highest heritability and largest sample size. The genetic correlations between MVP, 23andMe, UK Biobank and FinnGen were high (>0.71) despite these studies using a variety of phenotype definitions, e.g., structured interview-based clinical diagnosis of MDD, self-reported treatment or self-reported diagnosis. The consistency in collection for the large UKB and MVP samples reduced ascertainment heterogeneity within samples and likely increased study power. The genetic findings were replicated in an entirely independent sample of 1.3 million participants from 23andMe, where cases of depression were determined by participants’ answers to questions about having been diagnosed or treated for depression. These results demonstrated the consistency of GWAS findings once adequate power is achieved and the utility of different phenotyping methods even though they may reduce the specificity of findings for a core MDD phenotype [ 99 ].
This largest GWAS of MDD confirmed many previously reported loci and identified new ones. In addition to depression-related phenotypes, the most significant genetic correlation for MDD was tiredness/lethargy. The most significantly genetically correlated brain imaging phenotype was left subcallosal cortex gray matter volume. A tissue-based transcriptome-wide association study (TWAS) identified 153 genes in 14 tissues (13 brain regions plus whole blood). A follow-up variant prioritization that tested if the same locus is shared between GWAS and tissue-specific expression quantitative trait loci (eQTL) identified five gene–tissue pairs: CCDC71 –amygdala, FADS1 –cerebellar hemisphere, SPPL3 –frontal cortex, TRAF3 –hypothalamus and LAMB2 –whole blood. The protein product of CCDC71 and FADS1 is involved in the regulation of fatty acids and lipid metabolism. Several studies have been reported for increased risk of MDD due to depletion of omega-3 fatty acid, although the mechanism of how omega-3 supplementation treats depression is still unclear [ 99 , 104 ]. The protein product of SPPL3 (signal peptide peptidase-like 3) is involved in various cellular signaling cascades, including the T cell receptor signalling pathway and positive regulation of protein dephosphorylation [ 105 ]. The protein product of TRAF3 (TNF receptor-associated factor 3) controls the response of type-1 interferon, where studies have demonstrated an increased risk of MDD development associated with treatments based on interferon [ 106 ]. The protein product of LAMB2 (laminin subunit beta 2) is an integral part of neuromuscular junctions and is involved in neuropathic pains, a disturbance that influences the brain pathways in MDD [ 107 ]. Another top finding from the TWAS analysis was DRD2 (D2 dopamine receptor) with a significantly predicted decreased expression in the nucleus accumbens, which is a drug target and critical part of the mesolimbic dopamine reward circuit that has long been implicated in depression. The authors noted this finding from hypothesis-free investigations as “remarkable with respect to known biology” and provided optimism that novel genetic findings could help elucidate molecular mechanisms and highlight new therapeutic targets. Overall tissue expression analysis identified enrichment across all brain tissues and the pituitary, with the strongest findings for the frontal cortex Brodmann area (BA) 9, cortex, cerebellar hemisphere, anterior cingulate cortex, BA24 and cerebellum, and no enrichment in non-neuronal tissue. The most enriched biological processes were involved in nervous system development, synapse assembly, and organization. Gene-drug relationships were explored by drug mapping and identified four drugs that are either estrogen receptor agonists (diethylstilbestrol, implanon (etonogestrel implant)) or anti-estrogens (tamoxifen and raloxifene), suggesting opportunities for re-purposing, plus nicotine, cocaine, cyclothiazide, felbamate and riluzole. The latter three drugs have been shown to modulate glutamatergic activity, an interesting finding in the context of glutamate’s emerging role in mood disorders [ 99 ].
8. GWAS Identify Genetic Correlations between MDD and Other Disorders and Traits
GWAS have revealed the polygenic and pleiotropic nature of psychiatric disorders. There exists a high level of psychiatric comorbidity, and the heritability of psychiatric disorders based on twin studies ranges from ~40 to 80% [ 1 , 108 , 109 ]. Many casual variants are shared across these disorders and traits, as pleiotropy is pervasive [ 110 , 111 ]. Shared genetics between phenotypes can be calculated by genetic correlation, which is estimated from GWAS summary statistics using linkage disequilibrium score regression (LDSR). Initial GWAS based on large sample sizes highlighted that the strongest significant genetic correlations for MDD were with other major adult psychiatric disorders (schizophrenia and bipolar disorder (BD)), neuroticism, college completion and educational attainment, measures of sleep quality, coronary artery disease, triglycerides, body fat, waist-to-hip ratio, an earlier age of smoking initiation, and traits related to female reproductive life events (age at menarche and age at menopause; [ 4 , 5 ]). The most recent and largest GWAS reports significant genetic correlations for MDD with 668 diseases, disorders and traits from most categories of biobank-type phenotypes tested [ 99 ].
9. Combined Analysis of MDD with Brain-Related Phenotypes Increases Discovery of New Loci
Based on genetic correlations, some phenotypes have been co-analyzed with MDD to identify additional risk loci. A study of the genetics of the mood disorder spectrum meta-analyzing MDD and bipolar disorder GWAS data identified 73 genome-wide significant loci, 15 of which were novel for mood disorders. Genetic correlations revealed that type 2 bipolar disorder correlates strongly with recurrent and single episode major depressive disorder [ 112 ]. Interestingly, post-GWAS analyses identified both similarities and differences between the mood disorders. For example, cell types enriched for MDD-associated genes were also enriched for BD-associated genes (dopaminergic neuroblasts; dopaminergic, GABAergic and midbrain nucleus neurons from embryonic mice; interneurons; and medium spiny neurons), but some cell types enriched for BD-associated genes had no enrichment for MDD-associated genes (pyramidal cells from the CA1 region of the hippocampus and the somatosensory cortex, and striatal interneurons; [ 112 ].
Anxiety and stress-related disorders (ASRDs) have overlapping symptoms and high rates of comorbidity with MDD. There is a positive and significant genetic correlation between these disorders. The genetic correlation between the risk of anxiety disorder with major depressive disorder was found to be positive, at 0.83 ± 0.16, p = 1.97 × 10 –7 [ 113 ]. A cross-trait meta-analysis identified 5 pleiotropic loci simultaneously associated with MDD and ASRD, and highlighted NUP210L as a potential mediator of the genetic correlation between these disorders (GNOVA: rho = 0.59, se = 0.01, p = 5.32 × 10 –45 ) [ 114 ]. Cognitive impairment is a feature of MDD. Although there is a non-significant genetic correlation between general intelligence and MDD, GWAS analysis identified 92 shared loci between these two phenotypes, with 69 and 64 loci novel for MD and intelligence, respectively [ 115 ]. Here, there was a balanced mixture of directional effects among the loci shared between MDD and general intelligence, meaning that SNP alleles associated with increased risk of MDD are associated with lower intelligence at some loci and higher intelligence at other loci.
Late-onset Alzheimer’s disease (LOAD) exhibits comorbidity with MDD and anxiety, and MDD is associated with an increased risk of LOAD. Pleiotropy analysis found moderate enrichment for SNPs associated with LOAD across increasingly stringent levels of significance with the MDD GWAS association. Associated SNPs mapped to 40 genes, including 9 genes on chromosome 11 in the known LOAD-risk regions that contain the SPI1 gene and MS4A genes cluster, plus other novel pleiotropic risk-loci for LOAD conditional with MDD [ 116 ]. Attention deficit hyperactivity disorder (ADHD) in childhood or adolescence is associated with an increased risk of subsequent depression, and GWAS detects a genetic correlation between the two disorders ( r g = 0.52 (0.04)). Of 14 linkage disequilibrium-independent SNPs associated with ADHD and MDD separately and in a cross disorder meta-analysis (at genome-wide significance levels), 9 were novel findings for these disorders and revealed a molecular genetic basis for the overlap between disorders [ 117 ]. Cross-trait meta-analyses identified 89 genomic loci as being shared between MDD and insomnia, indicating a substantial shared genetic liability between these 2 interconnected conditions [ 118 ].
10. Exploring Causal Relationships
Genetic correlations between MDD and other disorders and traits may reflect the pleiotropic effects of genes and biological influences across both phenotypes but also may be a causal effect of MDD on other traits (for example, depression influencing sleep quality) or the causal effect of other phenotypes on MDD (for example, sleep quality leading to depression). Mendelian randomisation (MR) estimates causal relationships between genetically based traits by taking SNPs associated with one trait, the exposure, and using them as genetic instruments to test for causal effects of the exposure on a second trait, the outcome [ 119 ]. MR has identified a putative causal effect of body mass index, years of education and interleukin (IL)-6 levels on MDD, a putative causal effect of MDD on a smoking phenotype (ever versus never smoked), metabolic syndrome and its components (waist circumference, hypertension, triglycerides, high-density lipoprotein cholesterol), coronary artery disease, stroke, and inflammatory bowel disease, and bidirectional causal effects for MDD each of the following: schizophrenia, neuroticism, pain, osteoarthritis pain, insomnia and atopic diseases (asthma, hay fever, and eczema) [ 4 , 5 , 118 , 120 , 121 , 122 , 123 , 124 , 125 , 126 ]. The latter bidirectional results are consistent with a shared biological basis for MDD and these disorders and traits. Overall, these MR findings highlight different phenotypes as potentially targetable risk factors for the treatment of MDD and opportunities to reduce comorbidities if MDD can be successfully treated or prevented.
11. Rare Variant Analysis of MDD
An analysis of 488,366 participants from the UK Biobank identified 3 rare large (>100 kilobases (kb)) CNVs (1q21.1 duplication, Prader–Willi syndrome duplication, and 16p11.2 duplication) that were robustly associated with self-reported depression [ 127 ]. These CNVs have previously been implicated in neurodevelopmental disorders such as autism, intellectual disability and schizophrenia, again supporting shared etiology between MDD and these disorders. Patients with MDD have also been reported to carry significantly more short deletions (CNVs < 100 kb) than control subjects. These are primarily in intergenic regions, and no single CNV was associated at genome-wide significant levels [ 128 ].
NGS-based sequencing applications such as whole-exome sequencing (WES) and whole-genome sequencing (WGS) allow for the detection of rare and low-frequency sequence variants that may affect the risk of MDD [ 98 , 129 ]. A low-coverage WGS study of 5303 Chinese women with recurrent MDD (selected to reduce phenotypic heterogeneity) and 5337 controls by the CONVERGE consortium identified 2 risk variants: 1 near the SIRT1 gene and another in an intron of the LHPP gene [ 94 ]. Small-scale WES studies have not identified robustly associated loci for MDD [ 130 , 131 ]. Neither has the largest WES study to date using 22,886 cases and 176,486 controls from UK Biobank, but the mood disorder phenotype was based on having seen a psychiatrist for “nerves, anxiety, tension or depression” [ 132 ]. As was the case for GWAS, several iterations of study designs with an ever-increasing sample size will most likely be needed to find robustly associated rare sequence variants for MDD. The reward will be a more direct identification of risk genes based on the locations of causative variants, particularly if detected within exonic regions.
12. Challenges for Genetic Studies of MDD
In comparison to schizophrenia, where the critical inflection point for GWAS lay at 15,000 cases, after which the discovery of a new locus increased linearly at an average of 230 new cases per new locus [ 133 ], each new genome-wide significant locus for MDD takes considerably more effort. The inflection point for MDD was found at 75,000–100,000 cases, with the discovery of a new locus then increasing linearly at an average of 1500 new cases per new locus, reflecting the disorder’s lower heritability and higher prevalence in the population [ 95 , 134 ]. As larger samples have succeeded in finding new loci, the h 2 SNP from MDD GWAS that has had >100,000 cases remained in a range of 0.087–0.113 ( Figure 1 ), which is less than one-third of the h 2 estimated from twin or family studies [ 135 ]. The missing heritability may be explained by even larger GWAS, the discovery of rare variants via NGS methods, or it may be that twin and family studies have overestimated heritability [ 136 ].
The findings obtained from GWAS analysis can be used to estimate individual-level risk measures by estimating polygenic risk scores (PRSs) that attempt to predict the genetic liability of MDD in a set of individuals independent of the original GWAS. PRS aggregates the number of risk alleles an individual carries by weighting each variant by its effect size, thereby summarizing the large genotype matrix into a single variable per individual [ 96 ]. Nagelkerke’s R 2 is used to calculate the proportion of phenotypic variance explained on the liability scale using PRSs. Despite a large number of loci identified, the variance prediction capability of MDD is ultra-low (Nagelkerke’s R 2 of 1.5–3.2% on the liability scale [ 4 ]) in comparison to other psychiatric disorders such as schizophrenia (Nagelkerke’s R 2 of 7.7% on liability scale [ 137 ]). Therefore, MDD-based PRSs are not going to have any immediate clinical utility as a diagnostic tool. However, PRSs may have uses post-diagnosis of a mood disorder, for example, in discrimination between BD and MDD cases [ 138 ] and for treatment stratification [ 139 ].
A major limitation of PRSs is that they are highly sensitive to ethnic background and thus have the greatest predictive power where the discovery sample used for GWAS and the independent target sample have the same genetic ancestry [ 140 ]. GWAS has a major diversity problem, as most have used samples of European descent [ 141 ]. GWAS in more diverse samples is emerging, such as a study of 15,771 individuals with depression and 178,777 control participants of East Asian ancestry [ 142 ]. Here, several depression loci were not transferable between studies of cohorts of East Asian and European ancestry. Transancestry genetic correlations between the depression outcomes in these cohorts were moderate (max r = 0.558) and considerably lower than those reported for schizophrenia ( r = 0.98) [ 142 ]. Therefore, genetic findings for MDD may not transfer beyond the studied population. More diverse GWAS can allow for more accurate PRS profiling and help the fine mapping of associated loci based on differential LD patterns in different populations.
Many studies have sought to explore gene-environment interactions through investigations of known major environmental factors for MDD, such as stressful life events (e.g., childhood trauma). Results here have been inconsistent, with some data indicating that a higher h 2 SNP for MDD is evident among individuals reporting lifetime trauma exposure [ 143 ], whereas other data suggests that in the absence of environmental adversity, MDD may have a stronger genetic basis [ 112 ]. Using measures of early-life risk factors and sociodemographic variables from UK Biobank, gene-environment interactions were reported for childhood trauma and the Townsend Index of material deprivation, where the effect of depression-based PRS was enhanced in participants exposed to more adverse social/socioeconomic environments [ 144 ]. The study reported no significant interactions between the depression-based PRS and adulthood trauma, recent stressful life events and household income. As genetic studies progress and further biobanks come on-stream, ideally with greater longitudinal data, the scope for gene-environment studies of MDD will increase.
13. Summary
As predicted [ 133 ], GWAS of MDD have been successful despite the obstacles of moderate heritability, high prevalence, heterogeneity of genetic and non-genetic factors, and heterogeneity of samples. We now have a working knowledge, although incomplete, about many of the biological processes, cell types, tissues and druggable targets that are enriched for MDD risk genes. We are starting to understand the genetic relationship between MDD and many psychiatric and non-psychiatric disorders and traits. We are uncovering the complexity of MDD genetics in diverse populations. We are starting to test new genetic findings within the paradigm of gene-environment interactions. All this provides a foundation for the re-evaluation of previous hypotheses and the development and testing of new hypotheses about the molecular mechanisms for this multi-factorial disorder. Although a comprehensive guiding strategy for the application of genetics in the clinical management of MDD is still many years away, we can be confident that accelerating genetics and functional genomics research can contribute to precision medicine for this very common and hugely disabling disorder.
Author Contributions
Conceptualization, M.K. and D.W.M.; methodology, M.K.; software, M.K.; validation, M.K.; formal analysis, M.K.; investigation, M.K. and F.B.; resources, M.K. and D.W.M.; data curation, M.K.; writing—original draft preparation, M.K., F.B. and D.W.M.; writing—review and editing, A.u.R. and D.W.M.; visualization, M.K.; supervision, A.u.R. and D.W.M.; project administration, A.u.R. and D.W.M.; funding acquisition, M.K., A.u.R. and D.W.M. All authors have read and agreed to the published version of the manuscript.
This work was supported by a grant from the International Research Support Initiative Program of the Higher Education Commission (HEC) of Pakistan (1-8/HEC/HRD/2021/10952).
Institutional Review Board Statement
Data were directly downloaded from published studies and no additional ethics approval was needed. Each study is referenced and details on ethics approval are available in each manuscript.
Informed Consent Statement
Not applicable.
Data Availability Statement
Conflicts of interest.
The authors declare no conflict of interest.
Publisher’s Note: MDPI stays neutral with regard to jurisdictional claims in published maps and institutional affiliations.
Neurobiology of Depression
- First Online: 03 February 2022
Cite this chapter
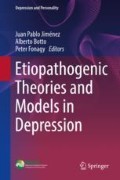
- Hernán Silva 7
Part of the book series: Depression and Personality ((DP))
698 Accesses
Hypotheses about the pathophysiology of depression have evolved over time. This chapter covers the most important findings in this regard. First, the classical monoamine hypothesis posited that depression is caused by an alteration in levels of one or more of the monoamines: serotonin, norepinephrine, and dopamine. More recently, research on the glutamatergic system has aroused great interest by examining the mechanism of action of ketamine, an N-methyl-D-aspartic acid (NMDA) receptor antagonist. Likewise, stressful life events can precipitate depressive episodes in vulnerable individuals. Abnormalities in the HPA axis have been associated with a hyperactive response to stress in depressed patients (the diathesis-stress model). Increased levels of inflammatory markers have been found in patients with depression and anti-inflammatory agents are being studied as antidepressants. Reduced production of BDNF and neuroplasticity can lead to depression. These pathophysiological mechanisms are reciprocally connected with each other. Major Depression is a heterogeneous entity and a variety of biological mechanisms may be involved.
This is a preview of subscription content, log in via an institution to check access.
Access this chapter
- Available as PDF
- Read on any device
- Instant download
- Own it forever
- Available as EPUB and PDF
- Compact, lightweight edition
- Dispatched in 3 to 5 business days
- Free shipping worldwide - see info
- Durable hardcover edition
Tax calculation will be finalised at checkout
Purchases are for personal use only
Institutional subscriptions
Amidfar, M., Woelfer, M., Réus, G. Z., Quevedo, J., Walter, M., & Kim, Y. K. (2019). The role of NMDA receptor in neurobiology and treatment of major depressive disorder: Evidence from translational research. Progress in Neuro-Psychopharmacology & Biological Psychiatry, 94 (Aug 30), 109668. https://doi.org/10.1016/j.pnpbp.2019.109668
Article Google Scholar
Bao, A. M., & Swaab, D. F. (2018). The human hypothalamus in mood disorders: The HPA axis in the center. IBRO Reports, 6 (Dec 14), 45–53. https://doi.org/10.1016/j.ibror.2018.11.008
Article PubMed PubMed Central Google Scholar
Bousman, C. A., Forbes, M., Jayaram, M., Eyre, H., Reynolds, C. F., Berk, M., Hopwood, M., & Ng, C. (2017). Antidepressant prescribing in the precision medicine era: A prescriber’s primer on pharmacogenetics tools. BMC Psychiatry, 17 (1), 60. https://doi.org/10.1186/s12888-017-1230-5
Casey, B. J., Craddock, N., Cuthbert, B. N., Hyman, S. E., Lee, F. S., & Ressler, K. J. (2013). DSM-5 and RDoC: Progress in psychiatry research? Nature Reviews Neuroscience. Nov, 14 (11), 810–814. https://doi.org/10.1038/nrn3621
Dantzer, R., O’Connor, J. C., Freund, G., Johnson, R. W., & Kelley, K. W. (2007). From information to sickness and depression: When the immune system subjugates the brain. Nature Reviews. Neuroscience, 9 , 45–56.
Google Scholar
Dean, J., & Keshavan, M. (2017). The neurobiology of depression: An integrated view. Asian Journal of Psychiatry. Jun, 27 , 101–111. https://doi.org/10.1016/j.ajp.2017.01.025
Ding, Y., & Dai, J. (2019). Advance in stress for depressive disorder. Advances in Experimental Medicine and Biology, 1180 , 147–178. https://doi.org/10.1007/978-981-32-9271-0_8
Article PubMed Google Scholar
Duman, R. S. (2009). Neuronal damage and protection in the pathophysiology and treatment of psychiatric illness: Stress and depression. Dialogues in Clinical Neuroscience, 11 (3), 239–255.
Duman, R. S., Shinohara, R., Fogaça, M. V., & Hare, B. (2019). Neurobiology of rapid-acting antidepressants: Convergent effects on GluA1-synaptic function. Molecular Psychiatry, 2019 Dec, 24 (12), 1816–1832. https://doi.org/10.1038/s41380-019-0400-x
Ferrari, F., & Villa, R. F. (2017). The neurobiology of depression: An integrated overview from biological theories to clinical evidence. Molecular Neurobiology. Sep, 54 (7), 4847–4865. https://doi.org/10.1007/s12035-016-0032-y
Gold, P. W. (2015). The organization of the stress system and its dysregulation in depressive illness. Molecular Psychiatry, Feb, 20 (1), 32–47. https://doi.org/10.1038/mp.2014.163
Gould, E., Tanapat, P., Rydel, T., & Hastings, N. (2000). Regulation of hippocampal neurogenesis in adulthood. Biological Psychiatry, 48 , 715–720.
Iadarola, N. D., Niciu, M. J., Richards, E. M., Vande Voort, J. L., Ballard, E. D., Lundin, N. B., Nugent, A. C., Machado-Vieira, R., & Zarate, C. A., Jr. (2015). Ketamine and other N-methyl-D-aspartate receptor antagonists in the treatment of depression: A perspective review. Therapeutic Advances in Chronic Disease. May, 6 (3), 97–114. https://doi.org/10.1177/2040622315579059
Jesulola, E., Micalos, P., & Baguley, I. J. (2018). Understanding the pathophysiology of depression: From monoamines to the neurogenesis hypothesis model - are we there yet? Behavioural Brain Research, 341 (Apr 2), 79–90. https://doi.org/10.1016/j.bbr.2017.12.025
Kanter, J. W., Busch, A. M., Weeks, C. E., & Landes, S. J. (2008). The nature of clinical depression: Symptoms, syndromes, and behavior analysis. Behavior Analyst, 31 (1), 1–21.
Lamers, F., Vogelzangs, N., Merikangas, K. R., de Jonge, P., Beekman, A. T., & Penninx, B. W. (2013). Evidence for a differential role of HPA-axis function, inflammation and metabolic syndrome in melancholic versus atypical depression. Molecular Psychiatry. Jun, 18 (6), 692–699. https://doi.org/10.1038/mp.2012.144
Leonard, B. E. (2001). Stress, norepinephrine and depression. Journal of Psychiatry & Neuroscience, 26 (Suppl), S11.
Liang, S., Wu, X., Hu, X., Wang, T., & Jin, F. (2018). Recognizing depression from the microbiota-gut-brain axis. International Journal of Molecular Sciences, 19 (6). https://doi.org/10.3390/ijms19061592
Liu, C. H., Zhang, G. Z., Li, B., Li, M., Woelfer, M., Walter, M., & Wang, L. (2019). Role of inflammation in depression relapse. Journal of Neuroinflammation . Apr 17 , 16 (1), 90. https://doi.org/10.1186/s12974-019-1475-7
Machado-Vieira, R., Salvadore, G., Diaz Granados, N., Ibrahim, L., Latov, D., Wheeler-Castillo, C., Baumann, J., Henter, I. D., & Zarate, C. A. (2010). New therapeutic targets for mood disorders. The Scientific World Journal, 10 , 713–726. https://doi.org/10.1100/tsw.2010.65
Miller, H. L., Delgado, P. L., Salomon, R. M., Berman, R., Krystal, J. H., Heninger, G. R., et al. (1996). Clinical and biochemical effects of catecholamine depletion on antidepressant-induced remission of depression. Archives of General Psychiatry, 53 , 117–128.
Monteleone, P., Serritella, C., Martiadis, V., & Maj, M. (2008). Decreased levels of serum brain-derived neurotrophic factor in both depressed and euthymic patients with unipolar depression and in euthymic patients with bipolar I and II disorders. Bipolar Disorders, 10 (1), 95–100. https://doi.org/10.1111/j.1399-5618.2008.00459.x
Pitsillou, E., Bresnehan, S. M., Kagarakis, E. A., Wijoyo, S. J., Liang, J., Hung, A., & Karagiannis, T. C. (2020). The cellular and molecular basis of major depressive disorder: Towards a unified model for understanding clinical depression. Molecular Biology Reports. Jan, 47 (1), 753–770. https://doi.org/10.1007/s11033-019-05129-3
Raison, C. L., Capuron, L., & Miller, A. (2006). Cytokines sing the blues: Inflammation and the pathogenesis of depression. Trends in Immunology, 27 , 24–31.
Ruhé, H. G., Mason, N. S., & Schene, A. H. (2007). Mood is indirectly related to serotonin, norepinephrine and dopamine levels in humans: A meta-analysis of monoamine depletion studies. Molecular Psychiatry, 12 , 331–359.
Sanacora, G., Treccani, G., & Popoli, M. (2012). Towards a glutamate hypothesis of depression: An emerging frontier of neuropsychopharmacology for mood disorders. Neuropharmacology, 62 , 63–77. https://doi.org/10.1016/j.neuropharm.2011.07.036
Steptoe, A., Hamer, M., & Chida, Y. (2007). The effects of acute psychological stress on circulating inflammatory factors in humans: A review and meta-analysis. Brain, Behavior, and Immunity, 21 (7), 901–912.
Villas Boas, G. R., Boerngen de Lacerda, R., Paes, M. M., Gubert, P., Almeida, W. L. D. C., Rescia, V. C., De Carvalho, P. M. G., De Carvalho, A. A. V., & Oesterreich, S. A. (2019). Molecular aspects of depression: A review from neurobiology to treatment. European Journal of Pharmacology . May 15 , 851 , 99–121. https://doi.org/10.1016/j.ejphar.2019.02.024
Willner, P., Scheel-Krüger, J., & Belzung, C. (2013). The neurobiology of depression and antidepressant action. Neuroscience and Biobehavioral Reviews, 37 (10 Pt 1), 2331–2371. Dec. https://doi.org/10.1016/j.neubiorev.2012.12.007
Woelfer, M., Kasties, V., Kahlfuss, S., & Walter, M. (2019). The role of depressive subtypes within the neuroinflammation hypothesis of major depressive disorder. Neuroscience, 403 , 93–110. https://doi.org/10.1016/j.neuroscience.2018.03.034 . Apr 1.
Wohleb, E. S., Franklin, T., Iwata, M., & Duman, R. S. (2016). Integrating neuroimmune systems in the neurobiology of depression. Nature Reviews Neuroscience. Aug, 17 (8), 497–511. https://doi.org/10.1038/nrn.2016.69
Download references
Author information
Authors and affiliations.
Department of Psychiatry, North Campus, Faculty of Medicine, University of Chile, Santiago, Chile
Hernán Silva
You can also search for this author in PubMed Google Scholar
Editor information
Editors and affiliations.
Department of Psychiatry and Mental Health East, Faculty of Medicine, University of Chile, Millennium Institute for Research in Depression and Personality (MIDAP), Santiago, RM, Chile
Juan Pablo Jiménez
Alberto Botto
Research Department of Clinical Educational and Health Psychology, University College London, London, UK
Peter Fonagy
Rights and permissions
Reprints and permissions
Copyright information
© 2021 Springer Nature Switzerland AG
About this chapter
Silva, H. (2021). Neurobiology of Depression. In: Jiménez, J.P., Botto, A., Fonagy, P. (eds) Etiopathogenic Theories and Models in Depression. Depression and Personality. Springer, Cham. https://doi.org/10.1007/978-3-030-77329-8_8
Download citation
DOI : https://doi.org/10.1007/978-3-030-77329-8_8
Published : 03 February 2022
Publisher Name : Springer, Cham
Print ISBN : 978-3-030-77328-1
Online ISBN : 978-3-030-77329-8
eBook Packages : Behavioral Science and Psychology Behavioral Science and Psychology (R0)
Share this chapter
Anyone you share the following link with will be able to read this content:
Sorry, a shareable link is not currently available for this article.
Provided by the Springer Nature SharedIt content-sharing initiative
- Publish with us
Policies and ethics
- Find a journal
- Track your research
Change Password
Your password must have 6 characters or more:.
- a lower case character,
- an upper case character,
- a special character
Password Changed Successfully
Your password has been changed
Create your account
Forget yout password.
Enter your email address below and we will send you the reset instructions
If the address matches an existing account you will receive an email with instructions to reset your password
Forgot your Username?
Enter your email address below and we will send you your username
If the address matches an existing account you will receive an email with instructions to retrieve your username

- April 01, 2024 | VOL. 181, NO. 4 CURRENT ISSUE pp.255-346
- March 01, 2024 | VOL. 181, NO. 3 pp.171-254
- February 01, 2024 | VOL. 181, NO. 2 pp.83-170
- January 01, 2024 | VOL. 181, NO. 1 pp.1-82
The American Psychiatric Association (APA) has updated its Privacy Policy and Terms of Use , including with new information specifically addressed to individuals in the European Economic Area. As described in the Privacy Policy and Terms of Use, this website utilizes cookies, including for the purpose of offering an optimal online experience and services tailored to your preferences.
Please read the entire Privacy Policy and Terms of Use. By closing this message, browsing this website, continuing the navigation, or otherwise continuing to use the APA's websites, you confirm that you understand and accept the terms of the Privacy Policy and Terms of Use, including the utilization of cookies.
The State of Our Understanding of the Pathophysiology and Optimal Treatment of Depression: Glass Half Full or Half Empty?
- Charles B. Nemeroff , M.D., Ph.D.
Search for more papers by this author
Major depressive disorder is a remarkably common and often severe psychiatric disorder associated with high levels of morbidity and mortality. Patients with major depression are prone to several comorbid psychiatric conditions, including posttraumatic stress disorder, anxiety disorders, obsessive-compulsive disorder, and substance use disorders, and medical conditions, including cardiovascular disease, diabetes, stroke, cancer, which, coupled with the risk of suicide, result in a shortened life expectancy. The goal of this review is to provide an overview of our current understanding of major depression, from pathophysiology to treatment. In spite of decades of research, relatively little is known about its pathogenesis, other than that risk is largely defined by a combination of ill-defined genetic and environmental factors. Although we know that female sex, a history of childhood maltreatment, and family history as well as more recent stressors are risk factors, precisely how these environmental influences interact with genetic vulnerability remains obscure. In recent years, considerable advances have been made in beginning to understand the genetic substrates that underlie disease vulnerability, and the interaction of genes, early-life adversity, and the epigenome in influencing gene expression is now being intensively studied. The role of inflammation and other immune system dysfunction in the pathogenesis of major depression is also being intensively investigated. Brain imaging studies have provided a firmer understanding of the circuitry involved in major depression, providing potential new therapeutic targets. Despite a broad armamentarium for major depression, including antidepressants, evidence-based psychotherapies, nonpharmacological somatic treatments, and a host of augmentation strategies, a sizable percentage of patients remain nonresponsive or poorly responsive to available treatments. Investigational agents with novel mechanisms of action are under active study. Personalized medicine in psychiatry provides the hope of escape from the current standard trial-and-error approach to treatment, moving to a more refined method that augurs a new era for patients and clinicians alike.
“Depression is the most unpleasant thing I have ever experienced…. It is that absence of being able to envisage that you will ever be cheerful again. The absence of hope. That very deadened feeling, which is so very different from feeling sad. Sad hurts but it’s a healthy feeling. It’s a necessary thing to feel. Depression is very different.”
—J.K. Rowling, interview, London Times , 2000
The well-known parable of the blind men and the elephant is the story of blind men who have never come across an elephant, and as each feels a different part of the elephant’s body, they describe the elephant based on their limited experience. None, of course, captures the totality of the animal. So it is with depression. There have been hundreds of volumes and many thousands of reports on various aspects of depression, ranging from nosology and epidemiology to pathophysiology and treatment. Each of these disciplines, applying, among other things, genetics, brain imaging, immunology, neurotransmitters and second messengers, neurotrophic factors, and animal models, like the blind men, “sees” parts of depression. Yet in spite of these efforts, our understanding of this major psychiatric disorder and its treatment remains limited.
Because so much has been published on the etiology and treatment of depression and yet there is so much that we do not know, I focus in this overview of the field on novel findings in all of the subdisciplines that comprise psychiatric investigation. By its very nature, this review cannot be comprehensive; rather, the major goal here is to provide an overview of where we are and where we need to go in order to attain our ultimate goals: an understanding of the pathogenesis of depression, which in turn will enhance our understanding of which of the many currently available evidence-based treatments will be most safe and effective in a given patient and aid us in the development of more effective treatments. What is most striking is the remarkable lack of concordance among researchers and clinicians on almost every single one of the major areas in the depression field. Indeed, there remains controversy about the appropriate approach and interpretation of the extant data regarding diagnosis, genomics, gene-by-environment interactions, animal models, mechanisms of action of evidence-based treatments, prediction of antidepressant efficacy and side effects (personalized medicine), efficacy and side effect burden of currently available treatments, and development of novel treatments. Perhaps this is not that different from other medical fields, such as oncology, neurology, and infectious disease, and it would appear to be similar to controversies in the posttraumatic stress disorder and schizophrenia fields.
Depression was recognized by Hippocrates (ca. 460–377 B.C.), Galen (ca. 129–199 A.D.), and Ishaq Ibn Imran (10th century A.D.), and these physicians’ early clinical descriptions well mirror those of today, including a profound loss of the capacity to feel pleasure, severe dysphoria (despondency), and a loss of will ( 1 ). These symptoms are similar to those of severe bereavement but occur in the absence of any clear precipitating event. Major depressive disorder is the focus of this discussion, and because of space constraints, there will be no coverage of bipolar depression, depression during pregnancy or the postpartum period, or childhood depression. The DSM-5 criteria ( 2 ), similar but not identical to those of ICD-10 ( 3 ), require the presence of five of nine well-known symptoms, including depressed mood, loss of pleasure or interest, significant appetite disturbance or body weight change, sleep disturbance, loss of energy, psychomotor changes, excessive guilt and/or worthlessness, decreased concentration, and recurring thoughts of death and/or suicide. Some common symptoms of major depression, such as diurnal mood variation and unexplained crying spells, are not included in the current diagnostic criteria. Further descriptors of major depression include levels of severity (mild, moderate, or severe) and certain features (psychotic or atypical features, seasonal pattern, melancholia). To be clear, unlike in other branches of medicine, this categorical or syndromal diagnosis of major depressive disorder rests entirely on descriptive phenomenology. Unlike the diagnosis and management of diabetes, which utilize HbA 1C levels, fasting blood glucose levels, and other metrics, the diagnosis of major depressive disorder has no validated biological markers that can serve as a validated ancillary diagnostic tool. Thus, unlike the use of EEG to document and augment clinical observations and patient history in the management of epilepsy, major depressive disorder relies entirely on patient self-report and clinician observation. Because epidemiology, clinical research, and clinical service delivery depend on this classification, and because two patients can meet criteria for major depressive disorder and have virtually no overlap in symptoms—for example, hypersomnia versus insomnia, decreased appetite versus increased appetite, suicidal ideation versus no suicidal ideation, and so on—the end result is a remarkable degree of heterogeneity in the diagnosis of major depressive disorder, an issue that has plagued the field. One group actually reported that some 1,500 DSM-IV symptom combinations can fulfill the diagnostic criteria for major depressive disorder! ( 4 ) It is clear that an 85-year-old patient with no prior history or family history of major depression who presents with many of the cardinal features of major depression is very different from a 30-year-old patient with a positive family history who presents with similar symptoms. This heterogeneity in the diagnosis of major depressive disorder is discussed further below in the context of research results in genomics, brain imaging, and treatment studies. Moreover, as others have pointed out ( 5 ), the low degree of reliability of the diagnosis of major depressive disorder in the DSM-5 field studies is a major concern, especially if all of the biological marker studies described below depend on this “gold standard.”
Several large-scale epidemiological studies using the DSM and ICD diagnostic classifications have revealed major depression to be remarkably common, as exemplified in the National Comorbidity Study Replication sample, with a 12-month prevalence rate of 6.6% and a lifetime prevalence rate of 16.2% ( 6 ). Similarly, data from the World Health Organization cite 12-month prevalence rates of 5.5%−5.9% and lifetime prevalence rates of 11.1%−14.6% ( 7 ). The average age at onset is 25 years. Women are twice as likely to suffer with depression, and the factors that contribute to this vulnerability remain obscure.
Another major source of disagreement in the field is how to deal with the substantial psychiatric comorbidity associated with the diagnosis of major depressive disorder. This includes both syndromal comorbidity and the presence of a variety of other psychiatric symptoms in patients with major depression. Indeed, comorbidity is more the rule than the exception, and this relationship is bidirectional. For example, the rate of major depression in patients with posttraumatic stress disorder (PTSD) is quite high, and a substantial proportion of patients with PTSD, particularly those with a history of early-life trauma, meet criteria for major depressive disorder ( 8 ). There are remarkably high levels of comorbidity of major depression in patients with syndromal anxiety disorders, such as social anxiety disorder and panic disorder—and indeed, prior to the development of DSM-5, an entire meeting was dedicated to determining whether generalized anxiety disorder exists as a freestanding diagnostic entity or is simply the forme fruste for major depression ( 9 ). The decision was made, based on the available evidence, to retain generalized anxiety disorder as a discrete diagnosis, but the controversy continues. Patients with obsessive-compulsive disorder (OCD) and substance use disorders also exhibit high prevalence rates of major depression. These comorbidities raise serious questions about the conduct of depression research. Should patients with comorbid psychiatric disorders be included in clinical studies of major depression, either pathophysiological or therapeutic? Because eliminating such patients to obtain a “pure” clinical sample of major depression effectively excludes a large proportion of the patients in clinical practice, any results obtained cannot be generalized to the overall depressed patient population.
Another important consideration is the course of major depression, which can be quite variable, ranging from patients who receive no treatment and spontaneously recover to those who develop chronic depression. What is clear is that for the majority of patients, recurrence rates are high, especially in the absence of continued treatment.
Finally, the age-old question of nature versus nurture in the etiology of major depression must be taken into account. As discussed below, it is now clear that a substantial risk for vulnerability to major depression is genetic, in the range of 35%−40% ( 10 ). The remainder of the risk is environmental, which includes a host of factors, including a history of childhood maltreatment, substance and alcohol abuse, more recent life stressors, social isolation, air pollution, socioeconomic status, and educational attainment ( 11 – 13 ). How these factors interact with genetic vulnerability to raise or lower the threshold for developing an episode of major depression is of great interest.
Medical Comorbidity
It has long been known that patients with certain common medical disorders exhibit rates of major depression that far exceed that of the general population. The prototype is, of course, patients with primary hypothyroidism: such patients are known to be nonresponsive to antidepressants and often show a return to euthymia with adequate thyroid hormone replacement or, if not, once euthyroid, do respond to antidepressant treatment ( 14 ). In addition, it is now clear that there is a bidirectional relationship between the hypothalamic-pituitary-thyroid axis and major depression, with patients with major depression exhibiting higher than expected rates of thyroid disease, most prominently symptomless autoimmune thyroiditis, as evidenced by the presence of antithyroid antibodies ( 15 ). There is now evidence that patients with major depression who have “high-normal” thyroid-stimulating hormone levels actually significantly benefit from thyroid hormone supplementation to achieve euthymia ( 16 ).
However, a host of other medical disorders present with inordinately high rates of comorbid major depression; these include certain cancers (even before diagnosis, most exemplified by pancreatic cancer); cardiovascular disease and stroke; diabetes; and, as demonstrated more recently, several autoimmune and inflammatory disorders, other CNS disorders such as multiple sclerosis, Parkinson’s disease, and Huntington’s disease, and chronic obstructive pulmonary disease ( 17 – 20 ). Recently, a common and understudied condition, burning mouth syndrome, a chronic oral pain disorder characterized by a generalized or localized burning sensation without the presence of any mucosal lesions, was found to be associated with high rates of major depression ( 21 ). A number of studies have documented the relatively poor treatment response in depressed patients with comorbid medical disorders, particularly in the elderly ( 22 ). It is important to note that several years ago, Schatzberg and his colleagues documented the high rate of pain symptoms in patients with major depression ( 23 ), and some have suggested that pain be added to the diagnostic criteria for major depressive disorder. The prominent comorbidity of major medical disorders, coupled with suicide, is the primary cause of the well-documented premature mortality in patients with major depression. Indeed, patients with depression die an average of 8 years earlier than comparable persons without depression ( 24 ). The underlying pathophysiological basis for the relationship between these major medical disorders and major depression remains largely obscure and understudied. There is some suggestion that inflammation may be one mechanism by which depression is associated with increased vulnerability to several of these disorders (see the section below on inflammation). Thus, it has been suggested that depression is a systemic illness that affects the brain and the body, with the latter effects associated with increased vulnerability to, and poor prognosis of, a number of medical disorders.
Animal Models of Depression
Similar to many of the other areas discussed in this review, this topic is also fraught with controversy. Let’s start with consideration of the fact that depression is a uniquely human condition. It simply does not occur in any other species. Although various animals, particularly nonhuman primates, are capable of exhibiting symptoms similar to depression in response to loss or if exposed to severe levels of stress, how these responses relate to the subjective experience of human depression is unclear. While animals in their natural habitat have been observed to exhibit some of the features of depression, the longitudinal nature of depression, including its recurrent course, is not apparent in animals. Similar to the relatively extreme measures necessary to induce rodents to consume alcohol, animal models of depression in rats or mice most often involve exposure to various types of stressors, including restraint stress, social isolation, electric foot shock, learned helplessness, chronic social defeat, olfactory bulbectomy, maternal deprivation, or chronic unpredictable stress ( 25 ). Such perturbations are out of the ordinary in the naturally occurring experiences of rodents in the wild. The results observed in such paradigms include a decrease in appetite, sexual behavior, and locomotor activity and an increase in assessed anxiety. Several of the cardinal features of major depression are impossible or virtually impossible to assess in rodents, including suicidality, decreased concentration, overwhelming guilt, and self-reproach. In addition to the models described above are pharmacological models used to induce depression, for example, the use of chronic corticosterone administration or serotonin depletion and genetic models that have bred generations of rodents for depressive symptoms ( 26 – 28 ). Another drawback of these models is that most of them have been limited to male rodents, a concern in view of the greater propensity for women to develop major depression. In spite of the clear differences between the syndrome of major depression and the phenotype of these animal models, a number of the models have face validity in predicting antidepressant activity of known and putative antidepressants. This has been both a curse and a blessing in that it has resulted in somewhat of a self-fulfilling prophecy; thus, if imipramine is effective in these models, new agents that test positive may well be “imipramine-like” and may therefore lead to the categorization of potentially useful new antidepressants that screen negative in these tests as being of no further interest.
One interesting novel approach has been the administration of exosomes from depressed patients to laboratory mice ( 29 ). Exosomes are small vesicles 40–100 nm in size that are released by many cell types, including neurons and glia. They contain a variety of molecules, including DNA, mRNA, and microRNA as well as proteins. One report ( 29 ) documented a microRNA, hsa-miR-139-5p, that is expressed differentially in exosomes of depressed patients compared with control subjects. When exosomes from these depressed patients were injected into normal mice, depressive-like behaviors were observed in several standard mouse depression models, including the forced swim test, tail suspension, and novelty-suppressed feeding. Moreover, treatment with exosomes from healthy control subjects or an antagonist of miR-139-5p blocked the depressogenic effects of the exosomes from depression patients in mice.
Genetics, Epigenetics, and Gene-Environment Interactions
This is a large literature, and I will only briefly discuss the major issues in the field. The seminal question remains that of understanding the discrepancy between the clear findings that approximately one-third of the risk for major depression is genetic and the absence of identification of the genetic substrates that mediate this risk. Genome-wide association studies (GWASs) have attempted, in relatively large samples, to identify the loci that confer risk for major depression. The results have largely been disappointing on two counts. First, in early studies, there appeared to be overlap in risk for major depression and both bipolar disorder and schizophrenia ( 30 ). Second, each of the gene variants (single-nucleotide polymorphisms [SNPs]) identified, although statistically significant because of the large number of subjects studied, alone exerts a very small effect in terms of vulnerability to major depression. Third, unlike schizophrenia and autism spectrum disorder, the identification of copy number variants or rare variants of large effect by exome sequencing in major depression has not been as robust as expected ( 31 ).
Although the early GWAS studies failed to detect any meaningful and specific depression-associated loci ( 32 ), more recent studies have been more successful. Howard and colleagues ( 33 ) meta-analyzed data on more than 807,553 individuals (246,363 case subjects and 561,190 control subjects) from three of the largest depression GWASs. They identified 102 independent variants, 269 genes, and 15 gene sets associated with depression, including some previously reported to be involved in synaptic structure and neurotransmission. A replication sample of more than 1.3 million individuals from 23andMe confirmed 87 of the 102 depression-associated variants. However, in that study there was again evidence of shared genetic components between depression and other psychiatric disorders, including anorexia nervosa, attention deficit hyperactivity disorder, schizophrenia, and bipolar disorder.
A breakthrough was clearly the identification of two loci for major depression in a study of 5,303 Han Chinese women with recurrent major depression and 5,337 control subjects ( 34 ). Prior to that study, there were no robustly replicated genetic loci identified in more than 9,000 study subjects. What was unique about this study was the selection of only women who had recurrent and severe major depression, a design that reduced phenotypic heterogeneity in an ethnically homogeneous population. The two genome-wide loci identified that conferred risk for major depression were both on chromosome 10, one near the Sirtulin 1 gene (SIRTI) and the other in an intron of the phosphorlysine phosphohistidine inorganic pyrophosphate phosphatase gene (LHPP). Further analysis of 4,509 cases of the most severe subtype of major depression, melancholia, yielded an increased genetic signal at the SIRTI locus. The success of this study was likely driven by several factors—the rigid inclusion criteria for major depressive disorder (recurrent cases only and exclusion of mild depression), study of women only, and limitation to an ethnically homogeneous population. This study and others raised a seminal question that has plagued the depression field in general and the depression genetics field in particular, namely, the use of minimal versus in-depth phenotyping. To state the two extremes, minimal phenotyping would be exemplified by the use of patient inclusion in the depression group as defined by the use of the term “depression” or the prescription of an antidepressant in an electronic medical record or in self-reports. In-depth phenotyping, which is by its very nature much more labor- and cost-intensive, would utilize categorical and dimensional measures to determine syndromal status and symptom severity (as well as comorbidity), such as the Structured Clinical Interview for DSM-5 or the Mini International Neuropsychiatric Interview for the former and any one of the many depression symptom severity scales, such as the Hamilton Depression Rating Scale (HAM-D) or the Montgomery-Åsberg Depression Rating Scale (MADRS) for the latter. Cai et al. ( 35 ) addressed this issue by comparing minimal phenotyping and strictly defined major depression in a GWAS. The genetic architecture of minimal phenotyping definitions of depression was clearly different from that of strictly defined major depression, the former enriched for nonspecific effects. The heritabilities of major depression defined by minimal phenotyping strategies were much lower than those of major depression defined by full DSM-5 criteria. Most importantly, a larger percentage of the genome contributes to the shared genetic liability between minimal phenotyping definitions of depression and other psychiatric conditions than between strictly defined major depression and other conditions. It is my hope that these data will finally put an end to the notion that moving away from strict phenotypic characterization of major depression could find utility in elucidating the biological basis of depression, including its genetic underpinnings. Surely, well-characterized patients with well-defined subtypes, such as atypical, psychotic, and melancholic, will help elucidate the pathophysiological differences among them.
Several future directions are evident from these and related findings, and many have recently been summarized ( 36 ). GWAS identifies genomic regions, not the underlying biological mechanisms. Because the effect sizes for each of the identified variants is small, care must be taken to pursue any individual locus, in view of the costs and difficulties in conducting functional studies. As noted above, the role of rare versus common variants has not been fully explored in major depression.
The seesaw of opinion related to gene-by-environment interaction studies and their utility in elucidating the pathogenesis of major depression has been confusing to the field. After a slew of single gene candidate studies were reported and subsequently not replicated in GWASs, they fell into disfavor. Of course, many of these “replications” suffered from the phenotyping quality issue described above as well as from relatively small sample sizes. These candidate gene studies were largely based on our understanding of the hypothesized underlying biology—for example, the corticotropin-releasing hormone (CRH receptor 1 [CRHR1]) polymorphism interaction with a history of child abuse and neglect appearing to result in increased vulnerability to major depression ( 37 ). I would suggest that such an approach is complementary to the polygenic risk score (PRS), which has largely supplanted it. I view this as a particularly important avenue of investigation because the GWAS findings explain only a small fraction of the heritability of major depression. I would suggest that this gap will be filled by understanding gene-by-environment interactions and the role of epigenetic mechanisms. I find it particularly interesting, in relation to depression, that gene-by-gene (epistasis) and gene-by-gene-by-environment interactions have been relatively ignored, although our group demonstrated the utility of the latter approach ( 38 ). In addition, others have suggested that neither individual GWASs nor meta-analytic combinations have been helpful in disclosing which genetic variants contribute to a particular phenotype, and therefore most of the missing heritability is latent in GWAS data, which may conceal intermediary phenotypes. The PGMRA web server for phenotype-genotype interactions and causal relations introduces the concept of phenomics, which could be applied to major depression ( 39 ). In the end, of course, what is likely of paramount importance is the effect of genomic variation and epigenetic mechanisms on gene expression, and ultimately effects on the expression of proteins. DNA methylation is one form of epigenetic regulation, and although not as advanced as the GWAS investigations, scrutiny of the methylome has now been undertaken in major depression. For example, Aberg et al. ( 40 ) recently published the first large-scale methylome-wide association study (MWAS) of 1,132 individuals with major depression and control subjects and 61 postmortem samples of Brodmann’s area (BA) 10 and in two postmortem replication samples (BA 10 and 25). Like GWAS results, this MWAS identified many depression-associated methylated CpGs with modest effects. Moreover, there was significant overlap in the depression-associated sites observed in blood and postmortem brain tissue. Of considerable interest is the recent report from the same group ( 41 ) in which blood samples from 581 patients with major depression were obtained at baseline for MWAS, and the results predicted future disease status 6 years later—that is, the presence or absence of a DSM-IV diagnosis of major depressive disorder—by calculating a methylation risk score, analogous to the PRS. The loci identified in the major depression sample overlapped with genes found in prior GWASs and included genes implicated in inflammation and autoimmune disease.
What has not been carefully scrutinized until recently is the interaction between critical environmental factors with genotype on DNA methylation. Recently Czamara et al. (unpublished data, January 2020) studied five independent cohorts totaling 1,074 individuals to determine the effects of child abuse and genotype on the methylome. Gene-by-child abuse interactions explained most variance in 80% of the DNA methylation sites, mapping to genes enriched in brain transcripts related to development and synaptic function. This underscores the importance of including genotyping in studies seeking to determine the effects of environmental factors on epigenetic marks.
Finally, a novel approach by Turecki’s group ( 42 ) is noteworthy. This group recently reported the results of a single-nucleus transcriptomics study in the dorsolateral prefrontal cortex of 17 male individuals with major depression and 17 matched control subjects. More than 80,000 nuclei were sampled and 26 cellular clusters were identified, and >60% showed differential gene expression between the groups. The largest effects were observed in deep-layer excitatory neurons and oligodendrocyte precursor cells. Such an approach allows for the identification of cell-specific gene dysregulation in major depression, a major advance over previous studies of postmortem brain homogenates.
We are surely in our infancy in understanding the relative roles of these genetic and epigenetic alterations on relevant molecular mechanisms that involve the synthesis of critical proteins, including receptors, transporters, and enzymes.
The Preeminent Role of Child Abuse and Neglect
In a sea of controversy and discordant findings, it is quite clear that there is almost universal agreement that childhood maltreatment, in the form of physical, sexual, and emotional abuse and neglect, is associated with a marked increase in risk for major depression ( 43 ). This finding has been replicated in a multitude of studies, and the data have been reviewed, including in large meta-analyses ( 44 ). Indeed, in many ways the emerging importance of early-life trauma in the pathophysiology of major depression has become the prototype for gene-by-environment interaction studies. For example, Peyrot et al. ( 45 ) conducted a study of 1,645 patients with major depression and 340 control subjects and determined that PRSs and childhood trauma independently affected major depression risk, but the effect of PRSs on depression was significantly increased in the presence of childhood trauma. Thus, patients with high PRSs and exposure to early-life trauma are at a particularly high risk for developing major depression. Moreover, it is now clear that patients with major depression with a history of early-life adversity exhibit a more virulent course of illness, including an earlier age at onset, more inpatient hospitalizations, more frequent suicide attempts and completed suicides, and relative resistance to evidence-based treatments, both psychopharmacology and psychotherapy ( 46 ). For example, in a recent study in France, Yrondi et al. ( 47 ) studied 256 patients with major depression and reported that there was a significant correlation between Childhood Trauma Questionnaire total score and MADRS and Quick Inventory of Depressive Symptomatology depression severity scores. More specifically, subscales of childhood sexual abuse and physical abuse were correlated with depression severity.
Although space constraints preclude a comprehensive discussion of this area, there is now a wealth of data on the manifold effects of childhood maltreatment on the CNS and on multiple physiological systems. In short, both laboratory animal studies and clinical studies have revealed long-lasting, persistent consequences of early-life adversity, including alterations in structural and functional brain imaging ( 48 ), immune function and inflammation, neuroendocrine axes, and the autonomic nervous system, to name just some of the findings ( 49 ). These profound effects are believed to mediate the shortened lifespan and increased vulnerability of victims of child abuse and neglect to a multitude of psychiatric and medical disorders, including major depression. However, these findings have other important implications. It is now unclear whether many of the biological alterations previously reported to occur in major depression are in fact actually a consequence of childhood maltreatment. This may be the case, for example, for the many reports of hypothalamic-pituitary-adrenal axis alterations in major depression, as well as the reports of reductions in the size of the hippocampus as assessed by structural MRI ( 50 , 51 ). Unfortunately, in the vast majority of studies that have sought to uncover biological alterations in major depression, assessment of childhood trauma was not included. Future studies, both of pathophysiology and treatment, will need to consider assessment of early-life adversity because of both its profound effects on a number of putative biomarkers and its negative effects on treatment response.
What Happened to the Monoamine Theory of Depression?
If I were writing this review 20 years ago, I would have related a tidy story about how three monoamine systems in the brain—serotonin, norepinephrine, and dopamine—are the major players in the pathophysiology of depression. The narrative would go something like this: Serotonin and norepinephrine circuits arise in the most ancient parts of the brain, in the raphe nuclei and the pons, respectively, and send widespread projections to the forebrain, where they exert control over a wide variety of physiological functions, many of which are awry in major depression, including appetite, libido, concentration, and mood. In addition, dopamine mediates the primary, perhaps pathognomonic, symptom of depression, namely, anhedonia. Depletion of these monoamines in laboratory animals with drugs such as reserpine or more selective agents that destroy serotonin or norepinephrine neurons leads to depressive-like symptoms in animals. Hundreds of published reports documented relative reductions of these neurotransmitters or their metabolites in CSF, blood, or urine of patients with major depression, and postmortem studies often supported these findings ( 52 ). Effective antidepressants were shown to act primarily on these circuits as reuptake inhibitors, thereby increasing the availability of these neurotransmitters in the synapse to further stimulate postsynaptic receptors. These observations, coupled with results of both depletion and provocative clinical studies such as the blunted growth hormone response to adrenergic and dopaminergic agonists in patients with major depression, supported these views ( 53 ). In those years, clinicians discussed patient symptoms as being a picture of a “dopaminergic” depression, with severe anhedonia and psychomotor retardation, or a “serotonergic” depression, with sleep disturbance and reduced libido.
Unfortunately, the monoamine theory as described has not stood up to close scrutiny. For example, reserpine, which depletes the brain of 95% of serotonin, dopamine, and norepinephrine, produces depression in only about 15% of subjects. If monoamines are that important in mood regulation, how can one walk around with <5% of one’s monoamines and be euthymic? Second, the pharmacological effects of selective serotonin reuptake inhibitors (SSRIs) and serotonin-norepinephrine reuptake inhibitors (SNRIs) as antagonists at the monoamine transporter sites are immediate, yet their antidepressant effects are delayed for 3–5 weeks, and even longer in some patients. Third, reappraisal of the biochemical marker studies noted above identified many negative studies that revealed no alterations in indices of activity of these monoamine circuits in depressed patients. Fourth, some antidepressants clearly do not act as reuptake inhibitors at these sites, including bupropion, agomelatine, ketamine, pimavanserin, and others. Fifth, even in previously untreated patients with major depression, SSRIs and SNRIs achieve remission in no more than 50% of the population. Sixth, recent studies by our group ( 54 ) have revealed no relationship between serotonin and norepinephrine transporter occupancy and treatment response in previously untreated patients with major depression who received escitalopram or duloxetine.
In spite of these “holes” in the monoamine theory, recent findings continue to implicate these systems in the pathophysiology of major depression. For example, in confirmation of a series of previous findings by Meyer and colleagues ( 55 ), Pizzagalli et al. ( 56 ) recently reported a reduction in dopamine transporter binding sites in 25 medication-free patients with major depression compared with 23 healthy control subjects, and these observations were confirmed in a postmortem study of 15 patients with major depression and 14 control subjects.
Another important and now repeatedly confirmed observation has been the increase in monoamine oxidase (MAO) B activity in the brains of patients with major depression, as assessed by positron emission tomography (PET). Moriguchi et al. ( 57 ) recently reported on 20 medication-free patients with major depression and 20 age-matched control subjects using a novel radioligand ([ 11 C]SL25.1188) to assess MAO-B activity. There was a marked increase in MAO-B activity in the patient group; 50% of the patients had MAO-B activity values in the prefrontal cortex higher than the highest levels in the control group.
In addition, there are many ongoing molecular pharmacology studies to determine the mechanism of action of antidepressants, going far beyond the monoamine transporter effects of these compounds. New studies have implicated the dopamine D 1 receptor ( 58 ) and the serotonin 5-HT 5A receptor ( 59 ), to name just two.
Brain Imaging Studies
At first glance, brain imaging studies would appear to be ideal to elucidate the pathophysiology of major depression. Structural and functional MRI, the latter with all its subdisciplines and related methodologies (e.g., diffusion tensor imaging), as well as MR spectroscopy and PET, have all been applied to the study of major depression. Indeed, as recounted recently by Spellman and Liston ( 60 ), more than 2,300 publications are now available in this area. The important question to ask, of course, is how to interpret the results of such studies. As regards the many reports of structural (volumetric) alterations in one or another CNS structure in patients with major depression, I would offer the following considerations. First, the effects are generally quite small. Second, meta-analyses have largely not provided widespread support for many of these findings. Third, and perhaps most important, what does a volumetric change in, for example, the hippocampus or prefrontal cortex actually mean? Is this due to a change in brain water? Dendritic or axonal atrophy? Neuronal degeneration? Ratio of glia to neurons? Cytoskeletal changes? No postmortem studies that I am aware of have correlated structural MRI findings with histopathology to address these questions.
In terms of functional brain imaging, largely fMRI studies, the literature is enormous and could not possibly be reviewed here. However, I must quote my colleague Daniel Weinberger, who, in cautioning on the interpretation of fMRI studies, said, “Have you ever read any published fMRI study of any psychiatric disorder that had no finding?” A number of caveats must be taken into account in critically reviewing these studies. First, in relation to resting-state fMRI, is the question of what the “resting state” represents. For anyone who has ever been in an MRI scanner, the “resting state” is hardly resting. Subjects are lying in the scanner thinking about a range of topics—their children, their job, when this will be over, etc. Despite this concern, leaders in the field such as Mayberg, Schatzberg, Liston ( 61 – 63 ), and others provided a solid framework by identifying increased functional connectivity in the subgenual anterior cingulate, thalamus, and default mode network, decreased functional connectivity in frontoparietal task control networks, and alterations in frontoparietal control and default mode networks in major depression. These findings, as reviewed by Spellman and Liston ( 60 ), have been replicated in meta-analyses ( 64 ). Most of these studies are cross-sectional in nature, but they represent a beginning framework for further study. They also offer the opportunity to serve as a target of novel therapeutic interventions (see below). It is important to determine whether the brain imaging observations described above are due wholly to the diagnosis of major depression or in part by—or with contributions from—a history of childhood maltreatment. As noted above, there is already considerable evidence that the previously reported reduction in hippocampal size in major depression is in fact due entirely to childhood maltreatment and is unrelated to the syndromal diagnosis of depression ( 50 , 51 ).
Another potential strength of brain imaging studies is the possibility of teasing out particular circuits that mediate specific depressive symptoms. One such recent example is the study by Rappaport et al. ( 65 ) in depressed adolescents in which a very discrete and incisive question was addressed, namely, What is the nature of reward circuitry in adolescence as a function of current and cumulative depression? The researchers found that current depression severity was associated with nucleus accumbens hypoactivity in response to the anticipation of a reward, whereas cumulative depression was associated with a blunted response to anticipation of reward in a cortico-striatal circuit. Such studies help to further refine our understanding of anhedonia in patients with major depression and provide a neuroanatomical basis for novel treatments.
In addition to providing the neurobiological basis for development of novel treatments such as deep brain stimulation, focused ultrasound, and novel forms of transcranial magnetic stimulation (see below), the major contribution of brain imaging studies may well lie in its serving as a component of the algorithm of personalized medicine in psychiatry to predict optimal response in an individual patient (see below).
The Immune System and Inflammation
Nearly 20 years ago, my colleagues Dominique Musselman and Andrew Miller led a study in which we reported that depressed patients and depressed patients with various cancers, but not cancer patients without depression or healthy control subjects, exhibited increased plasma concentrations of interleukin-6 (IL-6), a proinflammatory cytokine ( 66 ). We were following up on the pioneering studies by Maes and colleagues ( 67 ), who reported increases in inflammatory markers in depressed patients, as well as studies by Kronfol ( 68 ) and Schleifer et al. ( 69 ), who reported alterations in various immune measures in depressed patients, as well as our own studies of high rates of autoimmune thyroiditis in patients with major depression ( 15 ). An entire field of psychoimmunology has blossomed in the past two decades. In the space below, I attempt to summarize the major findings. I refer to recent reviews for comprehensive coverage, including one of our own ( 70 ). Alterations in both the adaptive and innate immune systems occur in major depression. It is now clear that major depression is associated with systemic immune activation, comprising alterations in inflammatory markers, immune cell numbers, and antibody titers. Multiple meta-analyses have confirmed the findings that proinflammatory cytokines and acute-phase proteins are increased in patients with major depression; the strongest evidence is for IL-6, tumor necrosis factor (TNF), and C-reactive protein (CRP). There is also evidence for an increase in inflammatory cytokine gene expression in peripheral blood mononuclear cells in major depression. However, not all patients with major depression exhibit this profile, and more recent studies have attempted to clinically characterize patients with major depression with this profile. There are now multiple reports of marked increases in inflammatory cytokine concentrations in blood and CSF in patients with prominent suicidality ( 71 ). It is important to note that elevations in inflammatory cytokines have also been reported in other major psychiatric disorders, including schizophrenia and bipolar disorder ( 72 ). Along with this consistent finding of an increase in inflammatory markers in major depression is the equally compelling but seemingly discordant finding that patients with major depression are relatively immunocompromised, as evidenced by a decreased lymphoproliferative response of T cells, decreased natural killer cell activity, and a decreased number of T helper cells. In our study of inflammatory markers ( 73 ), we found that never-treated patients with major depression exhibited increased cytokine production. In addition, exposure of plasma from the patients with major depression to peripheral blood mononuclear cells from healthy volunteers resulted in immunosuppression. It is important to note that in the large GWAS studies cited above, an inordinate number of the major depression loci identified were related to immune function, and these data have been recently reviewed ( 74 ). Lago et al. ( 75 ), in a study of 485 patients with major depression and 625 control subjects, discovered a SNP on the gene for the human CD300f immune receptor that alters its signaling and is associated with protection against major depression in women.
Of relevance is the question as to whether patients with major depression are more likely to be infected with bacteria or viruses when compared with the general population. There is considerable evidence that a past history of major depression is associated with an increased risk of infections, and this is generally interpreted to mean that patients with major depression are relatively immunosuppressed—and this in spite of the observed increase in proinflammatory cytokines. A related and relatively unexplored area is the relationship of major depression to autoimmune disease, alluded to earlier in this review. This bidirectional relationship is now well established. Patients with major depression have an increased risk of developing autoimmune diseases such as systemic lupus erythematosus, rheumatoid arthritis, autoimmune thyroiditis, multiple sclerosis, and irritable bowel syndrome, and patients with these disorders have high rates of major depression ( 76 ).
One of the major controversies that has plagued this field is the relative reliance on peripheral measures of inflammation, as opposed to measuring inflammation in the CNS, and the question of whether increased peripheral cytokines are in fact capable of producing CNS effects and playing a role in the pathogenesis of major depression. Recently Felger and colleagues ( 77 ) measured CRP and inflammatory cytokines in CSF and plasma from medication-free depressed patients. Their findings support the hypothesis that CRP is a peripheral biomarker that reflects both peripheral and central inflammation. In addition, several studies have now shown increases in both CSF and blood indices of inflammation in depressed patients, and, most importantly, PET ligands to measure inflammation in the CNS, such as expression of the translocator protein (TPSO), have provided concordant findings, although controversy continues as to what TPSO binding represents (microglial activation versus local myeloid cell or monocyte infiltration).
The evidence of CNS inflammation notwithstanding, there is considerable reason to believe that elevations in peripheral inflammatory cytokines exert effects on the CNS and may mediate depression associated with such states ( 78 ). It is important to note the many lines of evidence that support this view. First, peripheral cytokines can enter the CNS via the circumventricular organs in the brain, which contain fenestrated capillaries, unlike other regions protected by the blood-brain barrier. Second, there is evidence that cytokines can be transported across cerebral capillaries by a transport mechanism. Third, cytokines can bind to receptors on vagal afferents that project to the CNS. Fourth, treatment of human subjects or laboratory animals with alpha interferon (previously used to treat malignant melanoma and hepatitis C) results in a cytokine “storm” and a marked increase in depressive symptoms and suicidality. Finally (and discussed in more detail below, in the section on treatment), there is evidence that anti-inflammatory treatments, including TNF antagonists, which do not cross the blood-brain barrier, possess antidepressant properties, especially in patients with major depression with evidence of increased inflammation.
As in the discussion above on brain imaging, the role of childhood maltreatment in the inflammation observed in patients with major depression is of paramount consideration. There is much evidence, preclinical and clinical, that early-life trauma results in a long-lasting increase in proinflammatory cytokine secretion ( 79 ).
Treatments, Old and New
Unlike other serious mental disorders, for which we have a limited armamentarium, there are a multitude of evidence-based treatments for depression. There is overwhelming evidence that compared with placebo, antidepressant medications are effective treatments. Beginning with the MAO inhibitors and the tricyclic antidepressants in the late 1950s and 1960s, followed by fluoxetine, approved by the U.S. Food and Drug Administration (FDA) in 1987, a slew of other SSRIs (sertraline, paroxetine, citalopram, escitalopram, fluvoxamine), SNRIs (including duloxetine and venlafaxine), and, finally, a number of other compounds (bupropion, nefazodone, trazodone, mirtazapine, vortioxetine, reboxetine, agomelatine) were introduced, providing clinicians with much to choose from for initial monotherapy. All of these and others have been shown to be superior to placebo in the treatment of major depression and are approved by the FDA or its European counterpart.
The good news is that there are many FDA-approved antidepressants. The bad news is that monotherapy, even optimized by dosage and duration, results in remission in only a minority of patients. This is exemplified in the now classic open-label STAR*D (Sequenced Treatment Alternatives to Relieve Depression) trial, in which, as assessed by the HAM-D, only 28% of patients achieved remission with up to 40 mg of citalopram ( 80 ). In our randomized double-blind study of never-treated patients with major depression ( 81 ), approximately 50% of patients achieved remission after treatment with escitalopram, duloxetine, or cognitive-behavior therapy (CBT).
There is some controversy in the field about whether one antidepressant is more efficacious than another. With the exception of a series of studies that suggested that tricyclic antidepressants, and clomipramine in particular, were more effective than SSRIs ( 82 ) and meta-analyses that suggested that the SNRI venlafaxine was more effective than SSRIs ( 83 , 84 ), there is no compelling evidence that in groups of patients any one antidepressant has superior efficacy, and the FDA has never awarded status of greater efficacy to any single agent. As described below, considerable effort is currently being expended on attempting to identify biomarkers that will aid in the prediction of optimal treatment for patients with major depression—that is, which antidepressant will provide the best efficacy and side effect profile for the individual patient sitting in your office. Barring such a development, we are left with a trial-and-error approach.
The unmet needs in the treatment of major depression have led to two major research areas: the search for novel antidepressants that are not “me-too” drugs and the development of augmentation/combination strategies. I briefly describe some of the latest findings.
To some extent, the early development of MAO inhibitors and tricyclic antidepressants, followed by the SSRIs and SNRIs, bolstered the monoamine theories of major depression because, by one mechanism or another, they increased the synaptic availability of serotonin, norepinephrine, and/or dopamine. However, some of the follow-up agents had little direct effect on these systems. One such example is bupropion, which was suggested to be a norepinephrine and dopamine reuptake inhibitor, but the concentrations required to generate such effects were not attainable with standard clinical dosages ( 85 ), and its mechanism of action remains obscure. Similarly, other antidepressants, some not available in the United States, such as agomelatine and tianeptine, clearly do not act directly on monoamine neurons or their receptors, and others, such as nefazodone, mirtazapine, and mianserin, exert relatively weak effects.
These observations, coupled with the observations noted earlier that even SSRIs may not act primarily via these systems and the emergence of novel antidepressants that clearly have little or no effect on monoamine circuits, such as esketamine, raise fundamental questions as to the mechanism of action of antidepressants and the underlying pathophysiology of major depression.
In addition to antidepressants, evidence-based psychotherapies, most notably CBT and interpersonal psychotherapy, are clearly effective in the treatment of major depression ( 86 , 87 ), and there is some evidence of the efficacy of more psychodynamically based psychotherapies ( 88 ). There remains controversy about the relative efficacy of psychotherapy and antidepressants, with some arguing that they are equal and others suggesting that antidepressants are more effective in more severe depression ( 89 , 90 ).
In recent years, remarkable progress has been made in the development and optimization of somatic nonpharmacological treatments, including electroconvulsive therapy (ECT), repetitive transcranial magnetic stimulation (rTMS), vagal nerve stimulation (VNS), and deep brain stimulation (DBS), and a host of other modalities a bit further down the road in development, including direct current stimulation and focused ultrasound. I cannot possibly summarize this area and do it any justice. Suffice it to say that ECT is generally regarded as the most effective of all treatments, and there has never been a well-powered comparison of ECT with any antidepressant in major depression. ECT is not without its side effects, including short-term memory loss, but modifications in electrode placement have, to a considerable extent, reduced this troubling concern. ECT, VNS, and rTMS are all FDA-approved for the treatment of depression, and several ongoing studies and recent advances in the field are worth noting. First, in collaboration with the Centers for Medicare and Medicaid Services, a very large multisite 5-year study of the efficacy of VNS is being undertaken in severe treatment-resistant major depression. This study will answer a great many unanswered questions in the field and hopefully will result in third-party reimbursement for this invasive but potentially life-saving intervention. Advances in rTMS have been rapid in recent years, especially with the eye-opening results reported by Williams and colleagues ( 91 ) on the remarkable efficacy of accelerated theta-burst intermittent TMS delivered for 10 minutes every hour over 10 hours for 5 days directed at the precisely targeted dorsolateral prefrontal cortex in patients with extremely refractory major depression. Although it was an open study and clearly one that will need to be replicated in a sham-controlled design, the observed remission rate of >90% is nothing short of remarkable.
I would be remiss if I omitted mention of DBS. Pioneered by Mayberg and colleagues, early positive studies ( 92 ) were followed by industry-sponsored programs that were less successful. There are many reasons for such contrasting results, which can be said of many major depression trials—patient heterogeneity, electrode placement, and other patient and investigator variables. What is so important about DBS is that it was a therapy solidly based on the neurobiology of the disorder, whether focused on the subgenual cingulate cortex or the medial forebrain bundle. It is very difficult to accrue a patient population of sufficient size to accurately test the efficacy of this treatment. It is my hope that the work in this area continues.
In view of the failure of monotherapy to achieve remission in the majority of patients with major depression, a number of augmentation and combination strategies have been applied. Again, the clinician has much to choose from in treating a so-called treatment nonresponder/nonremitter or partial responder. Some of the strategies are monoamine based and are derived from preclinical observations, such as the use of lithium augmentation, which was first shown in rodents to potentiate the action of tricyclic antidepressants on serotonergic neurotransmission ( 93 ). Others are less grounded in basic neuropharmacology but have been shown to be efficacious in converting nonresponders to responders or remitters. These include a host of atypical antipsychotics, such as olanzapine, quetiapine, aripiprazole, brexpiprazole, risperidone, and others, some FDA-approved for this purpose ( 94 ). Other agents have also been reported to be effective in this regard, including thyroid hormone (T 3 ), pimavanserin (a serotonin inverse agonist), pramipexole (a D 2 /D 3 agonist), ketamine and esketamine, brexanolone, estrogen (in perimenopausal women), and an increasing number of psychedelic drugs, such as psilocybin ( 95 – 99 ). Then of course there are the combination therapies including SSRIs and other antidepressants, most notably bupropion, venlafaxine, or mirtazapine ( 100 ).
All of these strategies are backed by evidence that among patients with major depression who have failed to benefit from SSRI or SNRI monotherapy, some percentage will respond to augmentation or combination, but the effect sizes are relatively small. For example, in the placebo-controlled brexpiprazole augmentation trials, only about 25% of patients treated with brexpiprazole attained remission, compared with 10% with placebo ( 101 ). Frankly, none of the augmentation or combination therapies provide robust effects in patients who are nonresponsive or partially responsive to SSRIs or SNRIs, despite statistically significant results. Moreover, many of the augmentation strategies have significant side effects, ranging from the weight gain with certain atypical antipsychotics to the concerns of cost, drug abuse liability, and tachyphylaxis with esketamine ( 102 ). Of course, one should not omit the data related to the combination of antidepressants and evidence-based psychotherapy, for which our group and others have provided ample evidence ( 103 ), nor the combination of antidepressants and stimulation techniques such as TMS ( 104 ).
What is so puzzling about this admittedly confusing field is the wide range of pharmacological agents, with few properties in common, and some that would appear to be quite antagonistic, that have been reported to augment the action of antidepressants in partial responders. How we can reconcile, for example, the efficacy of atypical antipsychotics such as olanzapine, quetiapine, or risperidone, all of which are D 2 /5-HT 2 antagonists, and the efficacy of pramipexole, a D 2 /D 3 agonist? What do lithium, T 3 , pramipexole, atypical antipsychotics, and pimavanserin have in common? As discussed in the next section, we unfortunately have little to guide us as to which augmentation or combination therapy to choose for a given patient. Moreover, we know enough about the effects of antidepressants and psychotherapy on various brain circuits at least to conclude that it is unlikely that they share a common mechanism of action. All of this, of course, highlights our fundamental ignorance of the pathophysiology of major depression.
Before moving on to discuss personalized medicine, it is important to note that the treatment of patients with major depression with comorbid disorders, including anxiety disorders and PTSD, is a remarkably unexplored area, at least partly because such patients are excluded from most industry-sponsored studies. Some data are, however, available. We recently reviewed the major depression–PTSD treatment literature ( 105 ), and although it is somewhat limited, there is evidence of efficacy of combination pharmacotherapy and psychotherapy, as well as ECT.
Personalized Medicine in Depression Treatment
A number of the unmet needs described above in terms of diagnostic acuity and treatment response in major depression could readily be fulfilled by the maturation of personalized medicine in psychiatry. There are two fundamental components of personalized medicine: the identification of individuals who are at risk for a particular disorder and the identification of the most optimal treatment for individuals who suffer with the disorder. Personalized or precision medicine has emerged as an innovative approach for disease classification, research, and clinical practice. Fundamentally, this emerging field attempts to combine a number of unique characteristics of an individual patient, including their symptom complex, various biomarkers such as brain imaging, genomics/epigenetics, neuroendocrine and inflammatory measures, and environmental variables and lifestyle, in order to predict disease susceptibility, assist in diagnosis, and select the most effective treatments, maximizing the likelihood of remission and minimizing adverse effects ( 106 – 108 ). This approach has been remarkably successful in oncology ( 109 ), and there is no reason why it should not be equally successful in psychiatry. Unlike oncology, which has cancer histopathology as the sine que non for diagnosis, we are plagued with highly complex, heterogeneous presentations within our syndromal diagnostic classifications, as exemplified in major depression. I would suggest that in view of this, it is unlikely that any single biomarker or approach will provide the basis for reliable prediction of individual treatment response. This is exemplified by the failure of commercially available pharmacogenomic tests to predict either antidepressant efficacy or side effects, in spite of the many claims to the contrary. This has been reviewed by the APA Work Group on Biomarkers and Novel Treatments ( 110 ) and others ( 111 – 113 ) who have come to the same conclusion. Indeed, the largest randomized clinical trial of pharmacogenomics to predict antidepressant efficacy, the Genomics Used to Improve Depression Decisions (GUIDED) study, failed to meet its primary endpoint, a change in HAM-D score, and remarkably also failed to predict side effects ( 114 ). Studies of these commercially available tests are in general burdened by small sample size, lack of blinding, unusually low remission rates, and, most importantly, the fact that the algorithm used by each company is proprietary and therefore not evaluable by journal reviewers or clinicians. For reasons that are unclear, perhaps related to intellectual property, many promising genetic variations, such as SNPs that are reported to predict antidepressant treatment response by our group and others, such as FKBP5, CRH binding protein, GPR56/ADGRG1, and NET ( 115 – 117 ), are not included in any of the commercially available tests. Indeed, the FDA has issued warnings about the lack of scientific evidence underlying these tests and the dangers for patients who discontinue antidepressants after receiving a warning about potential adverse effects of a medication they are currently receiving, with potentially disastrous outcomes associated with subsequent relapse ( 118 ). In spite of my concern about the currently available pharmacogenomic tests, I have little doubt that pharmacogenomics, coupled with a variety of other types of data, will play an important role in the future in prediction of antidepressant response.
In addition to pharmacogenomics, the other two major areas that have been explored as putative indices of treatment response in major depression are brain imaging and EEG. Space constraints preclude any serious review of the former area, but it is clear that functional connectivity studies, including those noted in an earlier section and others, such as that based on the 1,000-patient International Study to Predict Optimized Treatment in Depression (iSPOT-D) ( 119 ), hold great promise. As regards EEG, as recently as 2019, the APA Work Group on Biomarkers and Novel Treatments reviewed the literature and concluded that the available evidence did not support EEG as a reliable predictor of antidepressant use ( 120 ).
However, two recent reports lend support to the notion that more advanced analytic techniques applied to EEG data provide strong support for rethinking this conclusion. Wu et al. ( 121 ) studied 309 patients with major depression in the Establishing Moderators and Biosignatures of Antidepressant Response for Clinical Care for Depression (EMBARC) study and identified predictions of sertraline response. This was confirmed in two replication samples. More recently, Zhdanov et al. ( 122 ), using a machine-learning approach in 122 patients treated with escitalopram, found that baseline EEG recordings identified the SSRI responders with accuracy of almost 80% (sensitivity and specificity of 67.3% and 91%, respectively). For those patients in whom EEG was obtained at week 2 of treatment, the prediction was even better.
A number of recent developments will all contribute to the eventual success of personalized medicine in psychiatry. These include the achievement of very-large-scale data collection, rendered easier by the often-criticized electronic medical record. The increasing use and availability of digital technology tools, especially wearables, which can collect objective and subjective data from patients, allow for multiple domains to be tracked continuously. Such measures can include heart rate and respiratory parameters, motor activity, and sleep architecture, to name a few. Such devices are being adopted in cardiology and endocrinology and will surely be incorporated into personalized medicine in psychiatry. The game-changer here is the development of machine learning, a form of artificial intelligence that is able to take hundreds, even thousands, of measures into a model that can predict outcomes. It has been used with great success in the financial sector, transportation, and social media and is now being applied in medicine. For example, machine learning has been applied with some success to the STAR*D phase I study and the Combining Medications to Enhance Depression Outcomes (COMED) study to identify remission with citalopram, escitalopram, and combined escitalopram-bupropion but not combined venlafaxine-mirtazapine ( 123 ). Similarly, machine-learning analysis of data from the Genome-Based Therapeutic Drugs for Depression (GENDEP) study resulted in prediction of response and remission to escitalopram and nortriptyline ( 124 ). What is now clearly emerging is the inclusion of multiple types of data, ranging from genomics to gene expression to early-life trauma to functional brain imaging, to enrich machine-learning models and come closer to real clinical utility. Already, reports are appearing in which inclusion of “multi-omics” coupled with other data, including metabolomics and other biomarkers, was found to significantly improve prediction accuracy for antidepressant treatment outcomes ( 125 ). Prediction of treatment response to CBT and other psychotherapies is also an active avenue of investigation, and it includes both genomics ( 126 ) and brain imaging approaches.
Conclusions
After a half century of research on the pathophysiology and treatment of major depression, it is remarkable how much we have learned and perhaps even more remarkable how much we do not know. Below I outline the major issues and unanswered questions.
The diagnosis of major depressive disorder remains fraught with difficulty because of the remarkable heterogeneity that is captured under this umbrella term. The definitions of response and remission are arbitrary, and serious questions remain about the utility of those measures. A HAM-D score of 7 or a MADRS score of 10 is not euthymia, in spite of these metrics currently being used to define remission. The definition of treatment-resistant depression is still not generally agreed upon, especially as regards how one defines an adequate treatment trial and the number of failed treatments that are required for such a classification. How to handle the common psychiatric comorbidities to major depression, such as PTSD, OCD, social anxiety disorder, and generalized anxiety disorder, in clinical research or practice remains unclear. Although the Research Domain Criteria approach proposed by the National Institutes of Mental Health (NIMH) seemed to hold promise, and it still may in research studies, as former NIMH director Thomas Insel noted, it has not changed clinical practice ( 127 ).
It is clear that only a minority of patients with major depression achieve remission with an adequate monotherapy trial. We must conclude, therefore, that our standard treatments remain suboptimal for many, and possibly most, patients. Augmentation strategies, of which there are many, are effective in some patients but also fraught with side effects (e.g., those associated with atypical antipsychotics and lithium).
The mechanism of action of antidepressants is unknown. In spite of intensive research in this area, none of the theories of antidepressant action have been substantiated, nor do they appear applicable to all antidepressants. This includes their action on monoamine circuits, neurogenesis, second messengers, or changes in gene expression. In addition, the mechanisms of action of ECT, TMS, and VNS as well as evidence-based psychotherapy remain obscure. Similarly, the mechanisms of action of the variety of augmentation strategies are equally elusive.
The holy grails of personalized medicine in depression, namely, the identification of those who are at risk for depression and the ability to choose the best and safest treatments for an individual patient, have not yet been achieved.
Much of the aforementioned shortcomings are secondary to our poor understanding of the pathophysiology of major depression. In spite of 40 years of research, with a multitude of “windows” into the brain, the fundamental etiology of major depression remains unknown. However, considerable advances have been made in genomics, epigenetics, inflammation, and environmental factors. Perhaps the brain has a limited repertoire of response to injury and major depression is a final common pathway—one that can be reached by multiple roads, as exemplified, for example, by major depression associated with hypothyroidism or hypogonadism.
The factors underlying the higher prevalence rates of major depression in women compared with men remain unknown.
Mechanistic studies of the high comorbidity rates of major depression and major medical disorders are sorely lacking. This is due in part to the fact that no National Institutes of Health component has taken ownership of this area, and therefore funding for this research is limited.
Dr. Nemeroff’s research is supported by NIMH grant MH-117293 and National Institute on Alcohol Abuse and Alcoholism grant AA-024933.
Dr. Nemeroff has served as a consultant for Acadia Pharmaceuticals, Axsome, Compass Pathways, EMA Wellness, Epiodyne, Gerson Lehrman Group, Intra-Cellular Therapies, Janssen Research and Development, Magnolia CNS, Magstim, Navitor Pharmaceuticals, Signant Health, Sophos, Sunovion Pharmaceuticals, Taisho Pharmaceutical, Takeda, TC MSO, and Xhale; he is a stockholder in AbbVie, Antares, BI Gen Holdings, Celgene, Corcept Therapeutics Pharmaceuticals Company, EMA Wellness, OPKO Health, Seattle Genetics, TC MSO, Trends in Pharma Development, and Xhale; he has served on scientific advisory boards for the American Foundation for Suicide Prevention, the Anxiety Disorders Association of America (ADAA), the Brain and Behavior Research Foundation, the Laureate Institute for Brain Research, Magnolia CNS, Signant Health, Skyland Trail, and Xhale; he has served on boards of directors for ADAA, Gratitude America, and Xhale Smart; he has income sources or equity of $10,000 or more from American Psychiatric Association Publishing, CME Outfitters, EMA Wellness, Intra-Cellular Therapies, Magstim, Signant Health, and Xhale; and he has patents on a method and devices for transdermal delivery of lithium (US 6,375,990B1), on a method of assessing antidepressant drug therapy via transport inhibition of monoamine neurotransmitters by ex vivo assay (US 7,148,027B2), and on compounds, compositions, methods of synthesis, and methods of treatment (CRF receptor binding ligand) (US 8,551,996B2).
1 Gillespie CF, Szabo S, Nemeroff CB : Unipolar depression , in Rosenberg’s Molecular and Genetic Basis of Neurological and Psychiatric Disease , 6th ed. Edited by Rosenberg R, Pasqual JM . Oxford, UK, Elsevier (in press) Google Scholar
2 American Psychiatric Association : Diagnostic and Statistical Manual of Mental Disorders , 5th ed (DSM-5). Washington, DC, American Psychiatric Association, 2013 Crossref , Google Scholar
3 World Health Organization: International Classification of Diseases and Related Health Problems, 10th revision, 5th ed. Geneva, World Health Organization, 2016 Google Scholar
4 Ostergaard SD, Jensen SOW, Bech P : The heterogeneity of the depressive syndrome: when numbers get serious . Acta Psychiatr Scand 2011 ; 124:495–496 Crossref , Medline , Google Scholar
5 Schatzberg AF : Scientific issues relevant to improving the diagnosis, risk assessment, and treatment of major depression . Am J Psychiatry 2019 ; 176:342–347 Link , Google Scholar
6 Kessler RC, Berglund P, Demler O, et al. : The epidemiology of major depressive disorder: results from the National Comorbidity Survey Replication (NCS-R) . JAMA 2003 ; 289:3095–3105 Crossref , Medline , Google Scholar
7 Bromet E, Andrade LH, Hwang I, et al. : Cross-national epidemiology of DSM-IV major depressive episode . BMC Med 2011 ; 9:90 Crossref , Medline , Google Scholar
8 Rosen V, Ortiz N, Nemeroff CB : Double trouble: treatment considerations for patients with comorbid PTSD and depression . Curr Treat Options Psychiatry (in press) Google Scholar
9 Martin EI, Nemeroff CB : The biology of generalized anxiety disorder and major depressive disorder: commonalities and distinguishing features , in Diagnostic Issues in Depression and Generalized Anxiety Disorder: Refining the Research Agenda for DSM-IV . Edited by Goldberg D, Kendler KS, Sirovatka PJ, et al. . Washington, DC, American Psychiatric Association Publishing, 2010 , pp 45–70 Google Scholar
10 Sullivan PF, Neale MC, Kendler KS : Genetic epidemiology of major depression: review and meta-analysis . Am J Psychiatry 2000 ; 157:1552–1562 Link , Google Scholar
11 Nemeroff CB : Paradise lost: the neurobiological and clinical consequences of child abuse and neglect . Neuron 2016 ; 89:892–909 Crossref , Medline , Google Scholar
12 Wymbs BT, McCarty CA, Mason WA, et al. : Early adolescent substance use as a risk factor for developing conduct disorder and depression symptoms . J Stud Alcohol Drugs 2014 ; 75:279–289 Crossref , Medline , Google Scholar
13 Gu X, Guo T, Si Y, et al. : Association between ambient air pollution and daily hospital admissions for depression in 75 Chinese cities . Am J Psychiatry 2020 ; 177:735–743 Link , Google Scholar
14 Nemeroff CB, Evans DL : Thyrotropin-releasing hormone (TRH), the thyroid axis, and affective disorder . Ann N Y Acad Sci 1989 ; 553:304–310 Crossref , Medline , Google Scholar
15 Nemeroff CB, Simon JS, Haggerty JJ Jr, et al. : Antithyroid antibodies in depressed patients . Am J Psychiatry 1985 ; 142:840–843 Link , Google Scholar
16 Cohen BM, Sommer BR, Vuckovic A : Antidepressant-resistant depression in patients with comorbid subclinical hypothyroidism or high-normal TSH levels . Am J Psychiatry 2018 ; 175:598–604 Link , Google Scholar
17 Currier MB, Nemeroff CB : Depression as a risk factor for cancer: from pathophysiological advances to treatment implications . Annu Rev Med 2014 ; 65:203–221 Crossref , Medline , Google Scholar
18 Nemeroff CB, Goldschmidt-Clermont PJ : Heartache and heartbreak: the link between depression and cardiovascular disease . Nat Rev Cardiol 2012 ; 9:526–539 Crossref , Medline , Google Scholar
19 Steffen A, Nübel J, Jacobi F, et al. : Mental and somatic comorbidity of depression: a comprehensive cross-sectional analysis of 202 diagnosis groups using German nationwide ambulatory claims data . BMC Psychiatry 2020 ; 20:142 Crossref , Medline , Google Scholar
20 Mulugeta A, Zhou A, King C, et al. : Association between major depressive disorder and multiple disease outcomes: a phenome-wide Mendelian randomisation study in the UK Biobank . Mol Psychiatry 2020 ; 25:1469–1476 Crossref , Medline , Google Scholar
21 Kim J-Y, Kim Y-S, Ko I, et al. : Association between burning mouth syndrome and the development of depression, anxiety, dementia, and Parkinson disease . JAMA Otolaryngol Head Neck Surg 2020 ; 145:561–569 Crossref , Google Scholar
22 Pratt LA, Druss BG, Manderscheid RW, et al. : Excess mortality due to depression and anxiety in the United States: results from a nationally representative survey . Gen Hosp Psychiatry 2016 ; 39:39–45 Crossref , Medline , Google Scholar
23 Ohayon MM, Schatzberg AF : Chronic pain and major depressive disorder in the general population . J Psychiatr Res 2010 ; 44:454–461 Crossref , Medline , Google Scholar
24 Archer G, Kuh D, Hotopf M, et al. : Association between lifetime affective symptoms and premature mortality . JAMA Psychiatry (Online ahead of print, April 8, 2020) Google Scholar
25 Ménard C, Hodes GE, Russo SJ : Pathogenesis of depression: insights from human and rodent studies . Neuroscience 2016 ; 321:138–162 Crossref , Medline , Google Scholar
26 Ma L, Shen Q, Yang S, et al. : Effect of chronic corticosterone-induced depression on circadian rhythms and age-related phenotypes in mice . Acta Biochim Biophys Sin (Shanghai) 2018 ; 50:1236–1246 Crossref , Medline , Google Scholar
27 Wang Q, Timberlake MA 2nd, Prall K, et al. : The recent progress in animal models of depression . Prog Neuropsychopharmacol Biol Psychiatry 2017 ; 77:99–109 Crossref , Medline , Google Scholar
28 Weiss JM, Cierpial MA, West CH : Selective breeding of rats for high and low motor activity in a swim test: toward a new animal model of depression . Pharmacol Biochem Behav 1998 ; 61:49–66 Crossref , Medline , Google Scholar
29 Wei Z-X, Xie G-J, Mao X, et al. : Exosomes from patients with major depression cause depressive-like behaviors in mice with involvement of miR-139-5p-regulated neurogenesis . Neuropsychopharmacology 2020 ; 45:1050–1058 Crossref , Medline , Google Scholar
30 Shohat S, Amelan A, Shifman S : Convergence and divergence in the genetics of psychiatric disorders from pathways to developmental stages . Biol Psychiatry (Online ahead of print, May 28, 2020) Google Scholar
31 Kendall KM, Rees E, Bracher-Smith M, et al. : Association of rare copy number variants with risk of depression . JAMA Psychiatry 2019 ; 76:818–825 Crossref , Medline , Google Scholar
32 Lee SH, Ripke S, Neale BM, et al. : Genetic relationship between five psychiatric disorders estimated from genome-wide SNPs . Nat Genet 2013 ; 45:984–994 Crossref , Medline , Google Scholar
33 Howard DM, Adams MJ, Clarke T-K, et al. : Genome-wide meta-analysis of depression identifies 102 independent variants and highlights the importance of the prefrontal brain regions . Nat Neurosci 2019 ; 22:343–352 Crossref , Medline , Google Scholar
34 CONVERGE consortium : Sparse whole-genome sequencing identifies two loci for major depressive disorder . Nature 2015 ; 523:588–591 Crossref , Medline , Google Scholar
35 Cai N, Revez JA, Adams MJ, et al. : Minimal phenotyping yields genome-wide association signals of low specificity for major depression . Nat Genet 2020 ; 52:437–447 Crossref , Medline , Google Scholar
36 Ormel J, Hartman CA, Snieder H : The genetics of depression: successful genome-wide association studies introduce new challenges . Transl Psychiatry 2019 ; 9:114 Crossref , Medline , Google Scholar
37 Bradley RG, Binder EB, Epstein MP, et al. : Influence of child abuse on adult depression: moderation by the corticotropin-releasing hormone receptor gene . Arch Gen Psychiatry 2008 ; 65:190–200 Crossref , Medline , Google Scholar
38 Ressler KJ, Bradley B, Mercer KB, et al. : Polymorphisms in CRHR1 and the serotonin transporter loci: gene x gene x environment interactions on depressive symptoms . Am J Med Genet B Neuropsychiatr Genet 2010 ; 153B:812–824 Medline , Google Scholar
39 Arnedo J, del Val C, de Erausquin GA, et al. : PGMRA: a web server for (phenotype x genotype) many-to-many relation analysis in GWAS . Nucleic Acids Res 2013 ; 41(Web Server issue):W142–W149 Crossref , Medline , Google Scholar
40 Aberg KA, Dean B, Shabalin AA, et al. : Methylome-wide association findings for major depressive disorder overlap in blood and brain and replicate in independent brain samples . Mol Psychiatry 2020 ; 25:1344–1354 Crossref , Medline , Google Scholar
41 Clark SL, Hattab MW, Chan RF, et al. : A methylation study of long-term depression risk . Mol Psychiatry 2020 ; 25:1334–1343 Crossref , Medline , Google Scholar
42 Nagy C, Maitra M, Tanti A, et al. : Single-nucleus transcriptomics of the prefrontal cortex in major depressive disorder implicates oligodendrocyte precursor cells and excitatory neurons . Nat Neurosci 2020 ; 23:771–781 Crossref , Medline , Google Scholar
43 Lippard ETC, Nemeroff CB : The devastating clinical consequences of child abuse and neglect: increased disease vulnerability and poor treatment response in mood disorders . Am J Psychiatry 2020 ; 177:20–36 Link , Google Scholar
44 Humphreys KL, LeMoult J, Wear JG, et al. : Child maltreatment and depression: a meta-analysis of studies using the Childhood Trauma Questionnaire . Child Abuse Negl 2020 ; 102:104361 Crossref , Medline , Google Scholar
45 Peyrot WJ, Milaneschi Y, Abdellaoui A, et al. : Effect of polygenic risk scores on depression in childhood trauma . Br J Psychiatry 2014 ; 205:113–119 Crossref , Medline , Google Scholar
46 Harkness KL, Bagby RM, Kennedy SH : Childhood maltreatment and differential treatment response and recurrence in adult major depressive disorder . J Consult Clin Psychol 2012 ; 80:342–353 Crossref , Medline , Google Scholar
47 Yrondi A, Aouizerate B, Bennabi D, et al. : Childhood maltreatment and clinical severity of treatment-resistant depression in a French cohort of outpatients (FACE-DR): one-year follow-up . Depress Anxiety 2020 ; 37:365–374 Crossref , Medline , Google Scholar
48 Popovic D, Ruef A, Dwyer D, et al. : Traces of trauma: a multivariate pattern analysis of childhood trauma, brain structure, and clinical phenotypes . Biol Psychiatry (Online ahead of print, May 26, 2020) Google Scholar
49 Parker J, Nemeroff CB: The long-term biological and clinical consequences of child abuse and neglect, in Stress Genetics, Epigenetics, Genomics (Handbook of Stress, vol 4). Edited by Fink G. Oxford, UK, Elsevier (in press) Google Scholar
50 Vythilingam M, Heim C, Newport J, et al. : Childhood trauma associated with smaller hippocampal volume in women with major depression . Am J Psychiatry 2002 ; 159:2072–2080 Link , Google Scholar
51 Botros M, Hodgins G, Nemeroff CB: The long-lasting neurobiological scars of early life stress: implications for the neurobiology of depression, in Neurobiology of Depression: Road to Novel Therapeutics. Edited by Quevedo J, Carvalho A, Zarate C. Oxford, UK, Elsevier, 2019, pp 111–122 Google Scholar
52 Gillespie CF, Nemeroff CB : Biology of depression , in Psychiatric and Metabolic Disorders: Research Synthesis and Clinical Translation . Edited by McIntyre RS, Konarski JZ . New York, Nova Science Publishers, 2008 , pp 147–172 Google Scholar
53 Pitchot W, Hansenne M, Gonzalez Moreno A, et al. : Effect of previous antidepressant therapy on the growth hormone response to apomorphine . Neuropsychobiology 1995 ; 32:19–22 Crossref , Medline , Google Scholar
54 Owens MJ, Dunlop B, Plott S, et al. : Estimates of serotonin or norepinephrine transporter occupancy do not predict antidepressant response in a 12 week trial . Neuropsychopharmacology 2014 ; 39:S460–S461 Google Scholar
55 Meyer JH, Krüger S, Wilson AA, et al. : Lower dopamine transporter binding potential in striatum during depression . Neuroreport 2001 ; 12:4121–4125 Crossref , Medline , Google Scholar
56 Pizzagalli DA, Berretta S, Wooten D, et al. : Assessment of striatal dopamine transporter binding in individuals with major depressive disorder: in vivo positron emission tomography and postmortem evidence . JAMA Psychiatry 2019 ; 76:854–861 Crossref , Google Scholar
57 Moriguchi S, Wilson AA, Miler L, et al. : Monoamine oxidase B total distribution volume in the prefrontal cortex of major depressive disorder: an [11C]SL25.1188 positron emission tomography study . JAMA Psychiatry 2019 ; 76:634–641 Crossref , Medline , Google Scholar
58 Shuto T, Kuroiwa M, Sotogaku N, et al. : Obligatory roles of dopamine D1 receptors in the dentate gyrus in antidepressant actions of a selective serotonin reuptake inhibitor, fluoxetine . Mol Psychiatry 2020 ; 25:1229–1244 Crossref , Medline , Google Scholar
59 Sagi Y, Medrihan L, George K, et al. : Emergence of 5-HT5A signaling in parvalbumin neurons mediates delayed antidepressant action . Mol Psychiatry 2020 ; 25:1191–1201 Crossref , Medline , Google Scholar
60 Spellman T, Liston C : Toward circuit mechanisms of pathophysiology in depression . Am J Psychiatry 2020 ; 177:381–390 Link , Google Scholar
61 Dunlop BW, Rajendra JK, Craighead WE, et al. : Functional connectivity of the subcallosal cingulate cortex and differential outcomes to treatment with cognitive-behavior therapy or antidepressant medication for major depressive disorder . Am J Psychiatry 2017 ; 174:533–545 Link , Google Scholar
62 Oathes DJ, Patenaude B, Schatzberg AF, et al. : Neurobiological signatures of anxiety and depression in resting-state functional magnetic resonance imaging . Biol Psychiatry 2015 ; 77:385–393 Crossref , Medline , Google Scholar
63 Drysdale AT, Grosenick L, Downar J, et al. : Resting-state connectivity biomarkers define neurophysiological subtypes of depression . Nat Med 2017 ; 23:28–38 Crossref , Medline , Google Scholar
64 Gray JP, Müller VI, Eickhoff SB, et al. : Multimodal abnormalities of brain structure and function in major depressive disorder: a meta-analysis of neuroimaging studies . Am J Psychiatry 2020 ; 177:422–434 Link , Google Scholar
65 Rappaport BI, Kandala S, Luby JL, et al. : Brain reward system dysfunction in adolescence: current, cumulative, and developmental periods of depression . Am J Psychiatry 2020 ; 177:754–763 Link , Google Scholar
66 Musselman DL, Miller AH, Porter MR, et al. : Higher than normal plasma interleukin-6 concentrations in cancer patients with depression: preliminary findings . Am J Psychiatry 2001 ; 158:1252–1257 Link , Google Scholar
67 Berk M, Williams LJ, Jacka FN, et al. : So depression is an inflammatory disease, but where does the inflammation come from? BMC Med 2013 ; 11:200 Crossref , Medline , Google Scholar
68 Kronfol Z : Immune dysregulation in major depression: a critical review of existing evidence . Int J Neuropsychopharmacol 2002 ; 5:333–343 Crossref , Medline , Google Scholar
69 Schleifer SJ, Keller SE, Bond RN, et al. : Major depressive disorder and immunity: role of age, sex, severity, and hospitalization . Arch Gen Psychiatry 1989 ; 46:81–87 Crossref , Medline , Google Scholar
70 Beurel E, Toups M, Nemeroff CB : The bidirectional relationship of depression and inflammation: double trouble . Neuron (Online ahead of print, June 10, 2020) Google Scholar
71 Brundin L, Bryleva EY, Thirtamara Rajamani K : Role of inflammation in suicide: from mechanisms to treatment . Neuropsychopharmacology 2017 ; 42:271–283 Crossref , Medline , Google Scholar
72 Müller N, Weidinger E, Leitner B, et al. : The role of inflammation in schizophrenia . Front Neurosci 2015 ; 9:372 Crossref , Medline , Google Scholar
73 Syed SA, Beurel E, Loewenstein DA, et al. : Defective inflammatory pathways in never-treated depressed patients are associated with poor treatment response . Neuron 2018 ; 99:914–924.e3 Crossref , Medline , Google Scholar
74 Barnes J, Mondelli V, Pariante CM : Genetic contributions of inflammation to depression . Neuropsychopharmacology 2017 ; 42:81–98 Crossref , Medline , Google Scholar
75 Lago N, Kaufmann FN, Negro-Demontel ML, et al. : CD300f immunoreceptor is associated with major depressive disorder and decreased microglial metabolic fitness . Proc Natl Acad Sci USA 2020 ; 117:6651–6662 Crossref , Medline , Google Scholar
76 Gonzalez R, Nemeroff CB : Inflammatory diseases and their psychiatric manifestations , in Textbook of Medical Psychiatry . Edited by Summergrad P, Silbersweig DA, Muskin PR, et al. . Washington, DC, American Psychiatric Association Publishing, 2020 , pp 229–263 Google Scholar
77 Felger JC, Haroon E, Patel TA, et al. : What does plasma CRP tell us about peripheral and central inflammation in depression? Mol Psychiatry 2020 ; 25:1301–1311 Crossref , Medline , Google Scholar
78 Nemeroff CB, Duman RA : Inflammation and psychiatric disorders . Psychiatr Ann 2015 ; 45:222–224 Crossref , Google Scholar
79 Grosse L, Ambrée O, Jörgens S, et al. : Cytokine levels in major depression are related to childhood trauma but not to recent stressors . Psychoneuroendocrinology 2016 ; 73:24–31 Crossref , Medline , Google Scholar
80 Trivedi MH, Rush AJ, Wisniewski SR, et al. : Evaluation of outcomes with citalopram for depression using measurement-based care in STAR*D: implications for clinical practice . Am J Psychiatry 2006 ; 163:28–40 Link , Google Scholar
81 Dunlop BW, Kelley ME, Aponte-Rivera V, et al. : Effects of patient preferences on outcomes in the Predictors of Remission in Depression to Individual and Combined Treatments (PReDICT) study . Am J Psychiatry 2017 ; 174:546–556 Link , Google Scholar
82 Danish University Antidepressant Group : Paroxetine: a selective serotonin reuptake inhibitor showing better tolerance, but weaker antidepressant effect than clomipramine in a controlled multicenter study . J Affect Disord 1990 ; 18:289–299 Crossref , Medline , Google Scholar
83 Nemeroff CB, Entsuah R, Benattia I, et al. : Comprehensive Analysis of Remission (COMPARE) with venlafaxine versus SSRIs . Biol Psychiatry 2008 ; 63:424–434 Crossref , Medline , Google Scholar
84 Thase ME, Entsuah AR, Rudolph RL : Remission rates during treatment with venlafaxine or selective serotonin reuptake inhibitors . Br J Psychiatry 2001 ; 178:234–241 Crossref , Medline , Google Scholar
85 Owens MJ, Morgan WN, Plott SJ, et al. : Neurotransmitter receptor and transporter binding profile of antidepressants and their metabolites . J Pharmacol Exp Ther 1997 ; 283:1305–1322 Medline , Google Scholar
86 Craighead WE, Craighead LW : Behavioral and cognitive-behavioral psychotherapy , in Handbook of Psychology, vol 8, Clinical Psychology . Edited by Stricker G, Widiger TA . Hoboken, NJ, John Wiley & Sons, 2003 , 279–299 Crossref , Google Scholar
87 Markowitz JC, Weissman MM : Interpersonal psychotherapy: principles and applications . World Psychiatry 2004 ; 3:136–139 Medline , Google Scholar
88 Driessen E, Hegelmaier LM, Abbass AA, et al. : The efficacy of short-term psychodynamic psychotherapy for depression: a meta-analysis update . Clin Psychol Rev 2015 ; 42:1–15 Crossref , Medline , Google Scholar
89 Sinyor M, Fefergrad M, Zaretsky A : Cognitive behavioural therapy or antidepressants for acute depression? BMJ 2015 ; 351:h6315 Crossref , Medline , Google Scholar
90 Boschloo L, Bekhuis E, Weitz ES, et al. : The symptom-specific efficacy of antidepressant medication vs cognitive behavioral therapy in the treatment of depression: results from an individual patient data meta-analysis . World Psychiatry 2019 ; 18:183–191 Crossref , Medline , Google Scholar
91 Cole EJ, Stimpson KH, Bentzley BS, et al. : Stanford Accelerated Intelligent Neuromodulation Therapy for treatment-resistant depression . Am J Psychiatry 2020 ; 177:716–726 Link , Google Scholar
92 Crowell AL, Riva-Posse P, Holtzheimer PE, et al. : Long-term outcomes of subcallosal cingulate deep brain stimulation for treatment-resistant depression . Am J Psychiatry 2019 ; 176:949–956 Link , Google Scholar
93 Blier P, De Montigny C : Short-term lithium administration enhances serotonergic neurotransmission: electrophysiological evidence in the rat CNS . Eur J Pharmacol 1985 ; 113:69–77 Crossref , Medline , Google Scholar
94 Zhou X, Keitner GI, Qin B, et al. : Atypical antipsychotic augmentation for treatment-resistant depression: a systematic review and network meta-analysis . Int J Neuropsychopharmacol 2015 ; 18:pyv060 Crossref , Medline , Google Scholar
95 DeOreo E, Nemeroff CB : The hypothalamic-pituitary-thyroid axis and depression , in International Encyclopedia of Depression . Edited by Ingram RE . New York, Springer, 2009 , pp 336–339 Google Scholar
96 Fava M, Dirks B, Freeman MP, et al. : A phase 2, randomized, double-blind, placebo-controlled study of adjunctive pimavanserin in patients with major depressive disorder and an inadequate response to therapy (CLARITY) . J Clin Psychiatry 2019 ; 80:e1–e8 Crossref , Google Scholar
97 Fawcett J, Rush AJ, Vukelich J, et al. : Clinical experience with high-dosage pramipexole in patients with treatment-resistant depressive episodes in unipolar and bipolar depression . Am J Psychiatry 2016 ; 173:107–111 Link , Google Scholar
98 Sanacora G, Frye MA, McDonald W, et al. : A consensus statement on the use of ketamine in the treatment of mood disorders . JAMA Psychiatry 2017 ; 74:399–405 Crossref , Medline , Google Scholar
99 Reiff CM, Richman EE, Nemeroff CB, et al. : Psychedelics and psychedelic-assisted psychotherapy . Am J Psychiatry 2020 ; 177:391–410 Link , Google Scholar
100 Farah A, Blier P : Combination strategies for the pharmacological treatment of major depressive disorder , in American Psychiatric Publishing Textbook of Mood Disorders , 2nd ed. Edited by Nemeroff CB, Strakowski S, Rasgon N, et al. . American Psychiatric Association Publishing (in press) Google Scholar
101 Thase ME, Youakim JM, Skuban A, et al. : Efficacy and safety of adjunctive brexpiprazole 2 mg in major depressive disorder: a phase 3, randomized, placebo-controlled study in patients with inadequate response to antidepressants . J Clin Psychiatry 2015 ; 76:1224–1231 Crossref , Medline , Google Scholar
102 Horowitz MA, Moncrieff J : Are we repeating mistakes of the past? A review of the evidence for esketamine . Br J Psychiatry (Online ahead of print, May 27, 2020) Google Scholar
103 Dunlop BW, LoParo D, Kinkead B, et al. : Benefits of sequentially adding cognitive-behavioral therapy or antidepressant medication for adults with nonremitting depression . Am J Psychiatry 2019 ; 176:275–286 Link , Google Scholar
104 Schlaepfer TE, Kosel M, Nemeroff CB : Efficacy of repetitive transcranial magnetic stimulation (rTMS) in the treatment of affective disorders . Neuropsychopharmacology 2003 ; 28:201–205 Crossref , Medline , Google Scholar
105 Flory JD, Yehuda R : Comorbidity between post-traumatic stress disorder and major depressive disorder: alternative explanations and treatment considerations . Dialogues Clin Neurosci 2015 ; 17:141–150 Crossref , Medline , Google Scholar
106 Perna G, Nemeroff CB : Moving from precision to personalized psychiatry: clinical perspectives on the new era , in Precision Psychiatry . Edited by Williams LM, Hack LM . Washington, DC, American Psychiatric Association Publishing (in press) Google Scholar
107 Sanacora G : Is this where we stand after decades of research to develop more personalized treatments for depression? JAMA Psychiatry 2020 ; 77:560–562 Crossref , Google Scholar
108 Fernandes BS, Williams LM, Steiner J, et al. : The new field of “precision psychiatry” . BMC Med 2017 ; 15:80 Crossref , Medline , Google Scholar
109 Le Tourneau C, Kamal M, Bièche I : Precision medicine in oncology: what is it exactly and where are we? Per Med 2018 ; 15:351–353 Crossref , Medline , Google Scholar
110 Zeier Z, Carpenter LL, Kalin NH, et al. : Clinical implementation of pharmacogenetic decision support tools for antidepressant drug prescribing . Am J Psychiatry 2018 ; 175:873–886 Link , Google Scholar
111 Goldberg JF, Nemeroff CB : Pharmacogenomics and antidepressants: efficacy and adverse drug reactions , in Psychiatry Genomics . Edited by Tsermpini E, Patrinos GP, Alda M . Cambridge, Mass, Academic Press (in press) Google Scholar
112 Zubenko GS, Sommer BR, Cohen BM : On the marketing and use of pharmacogenetic tests for psychiatric treatment . JAMA Psychiatry 2018 ; 75:769–770 Crossref , Medline , Google Scholar
113 Smith TL, Nemeroff CB : To test or not to test: pharmacogenomics and depression . Br J Psychiatry 2020 ; 42:116–117 Crossref , Google Scholar
114 Greden JF, Parikh SV, Rothschild AJ, et al. : Impact of pharmacogenomics on clinical outcomes in major depressive disorder in the GUIDED trial: a large, patient- and rater-blinded, randomized, controlled study . J Psychiatr Res 2019 ; 111:59–67 Crossref , Medline , Google Scholar
115 Binder EB, Salyakina D, Lichtner P, et al. : Polymorphisms in FKBP5 are associated with increased recurrence of depressive episodes and rapid response to antidepressant treatment . Nat Genet 2004 ; 36:1319–1325 Crossref , Medline , Google Scholar
116 Belzeaux R, Gorgievski V, Fiori LM, et al. : GPR56/ADGRG1 is associated with response to antidepressant treatment . Nature Commun 2020 ; 11:1635 Google Scholar
117 Yoshida K, Takahashi H, Higuchi H, et al. : Prediction of antidepressant response to milnacipran by norepinephrine transporter gene polymorphisms . Am J Psychiatry 2004 ; 161:1575–1580 Link , Google Scholar
118 US Food and Drug Administration: Jeffrey Shuren, MD, JD, director of the FDA’s Center for Devices and Radiological Health and Janet Woodcock, M.D., director of the FDA’s Center for Drug Evaluation and Research on agency’s warning to consumers about genetic tests that claim to predict patients’ responses to specific medications. November 1, 2018 ( https://www.fda.gov/news-events/press-announcements/jeffrey-shuren-md-jd-director-fdas-center-devices-and-radiological-health-and-janet-woodcock-md ) Google Scholar
119 Goldstein-Piekarski AN, Staveland BR, Ball TM, et al. : Intrinsic functional connectivity predicts remission on antidepressants: a randomized controlled trial to identify clinically applicable imaging biomarkers . Transl Psychiatry 2018 ; 8:57 Crossref , Medline , Google Scholar
120 Widge AS, Bilge MT, Montana R, et al. : Electroencephalographic biomarkers for treatment response prediction in major depressive illness: a meta-analysis . Am J Psychiatry 2019 ; 176:44–56 Link , Google Scholar
121 Wu W, Zhang Y, Jiang J, et al. : An electroencephalographic signature predicts antidepressant response in major depression . Nat Biotechnol 2020 ; 38:439–447 Crossref , Medline , Google Scholar
122 Zhdanov A, Atluri S, Wong W, et al. : Use of machine learning for predicting escitalopram treatment outcome from electroencephalography recordings in adult patients with depression . JAMA Netw Open 2020 ; 3:e1918377 Crossref , Medline , Google Scholar
123 Chekroud AM, Gueorguieva R, Krumholz HM, et al. : Reevaluating the efficacy and predictability of antidepressant treatments: a symptom clustering approach . JAMA Psychiatry 2017 ; 74:370–378 Crossref , Medline , Google Scholar
124 Iniesta R, Hodgson K, Stahl D, et al. : Antidepressant drug-specific prediction of depression treatment outcomes from genetic and clinical variables . Sci Rep 2018 ; 8:5530 Crossref , Medline , Google Scholar
125 Athreya AP, Neavin D, Carrillo-Roa T, et al. : Pharmacogenomics-driven prediction of antidepressant treatment outcomes: a machine-learning approach with multi-trial replication . Clin Pharmacol Ther 2019 ; 106:855–865 Crossref , Medline , Google Scholar
126 Jiménez JP, Botto A, Herrera L, et al. : Psychotherapy and genetic neuroscience: an emerging dialog . Front Genet 2018 ; 9:257 Crossref , Medline , Google Scholar
127 Star neuroscientist Tom Insel leaves the Google-spawned Verily for … a startup? Wired, May 11, 2017 ( https://www.wired.com/2017/05/star-neuroscientist-tom-insel-leaves-google-spawned-verily-startup/ ) Google Scholar
- Roger S. McIntyre , M.D.
- Ned H. Kalin , M.D.
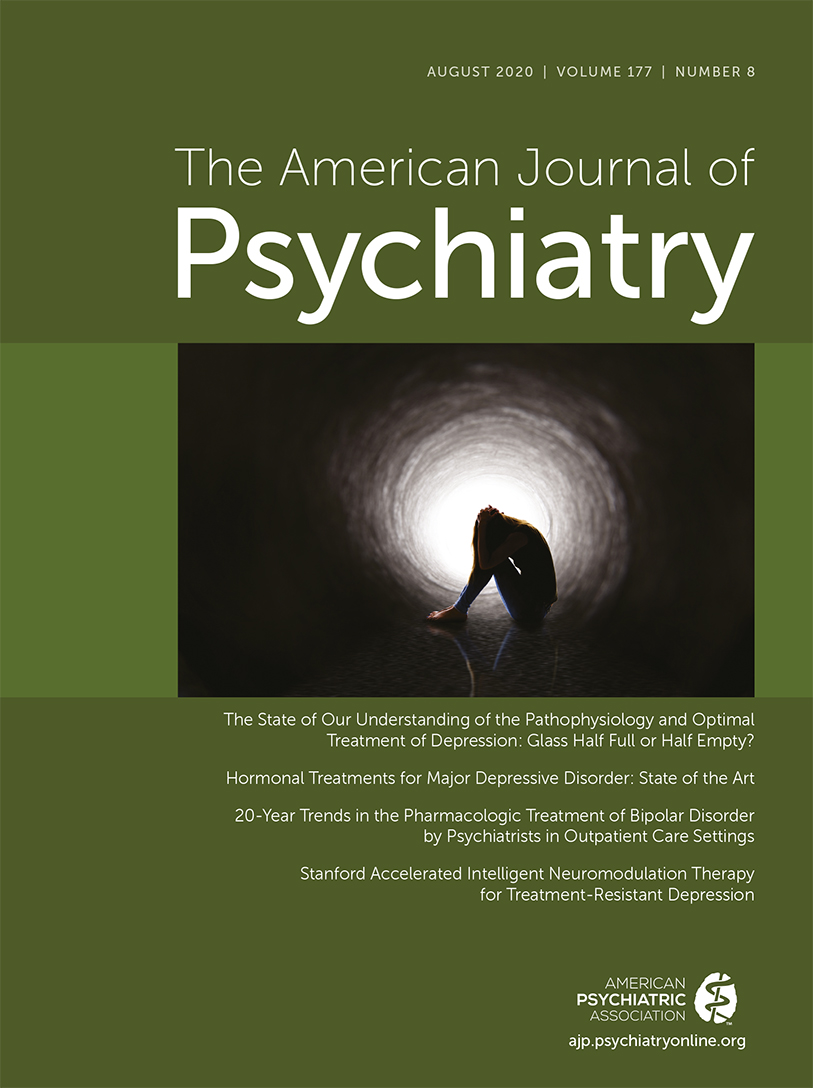
- Major Depressive Disorder
- - Google Chrome
Intended for healthcare professionals
- Access provided by Google Indexer
- My email alerts
- BMA member login
- Username * Password * Forgot your log in details? Need to activate BMA Member Log In Log in via OpenAthens Log in via your institution

Search form
- Advanced search
- Search responses
- Search blogs
- News & Views
- Antidepressants and...
Antidepressants and the serotonin hypothesis of depression
- Related content
- Peer review
- Tony Kendrick , professor of primary care 1 ,
- Susan Collinson , specialist TB case worker 2
- 1 Primary Care, Population Sciences and Medical Education, University of Southampton, Aldermoor Health Centre, Southampton, UK
- 2 Homerton University Hospital NHS Foundation Trust, London, UK
- Correspondence to: T Kendrick A.R.Kendrick{at}Southampton.ac.uk
Antidepressants remain an effective treatment for depression, even without the “chemical imbalance” explanation
A recent umbrella review of evidence for the serotonin theory of depression 1 was widely reported in UK media as showing that depression is not caused by low levels of serotonin or a “chemical imbalance” and therefore casting doubt on the use of selective serotonin reuptake inhibitor (SSRI) antidepressants by millions of people. 2 3 4 5
The review brought together existing systematic reviews, meta-analyses, and large dataset analyses on associations between depression and concentrations of serotonin and its metabolite 5-hydroxyindoleacetic acid (5-HIAA) in body fluids; serotonin 5-HT1A receptor binding; serotonin transporter (SERT) levels measured by imaging or postmortem analysis; tryptophan depletion; SERT gene polymorphism; and SERT gene-environment interactions. It reported no consistent evidence to support the hypothesis that depression is caused by reduced serotonin activity, and called for acknowledgment that the theory is not empirically substantiated. 1
The polarising debate that ensued risks undermining the evidence based treatment of depression and causing harm to people who take or need SSRI antidepressants. Critics of the review and its coverage noted that study selection was incomplete, as an omitted 2021 meta-analysis had concluded that changes in blood biochemistry, notably of L-tryptophan, were associated with depression. 6 The umbrella review was dismissed as nothing new and limited because peripheral and indirect measures of serotonin concentration or activity tell us nothing about activity at receptors between neurons in the brain. 7 Psychiatrists argued that use of SSRIs is not based on the simplistic theory that low serotonin causes depression but on clinical trial evidence. 6 7
Others, however, including the review’s lead author, interpreted the findings to imply that antidepressants do not work, suggesting they are barely distinguishable from placebos and may just numb emotions. 8 9 These contentions are not supported by evidence, went beyond the findings of the review, and were not expressed in its conclusions. 1 They could encourage sudden antidepressant cessation, causing withdrawal symptoms and risking relapse.
Public reaction on social media included fear, guilt, and feeling stigmatised for taking antidepressants on the one hand, and anger at experts’ dismissal of the legitimate concerns of patients about medication on the other.
Clinical response
How should patients and clinicians navigate these challenges? First and foremost, good evidence from randomised controlled trials shows that antidepressants are effective in treating people with new episodes of both less severe and more severe depression, 10 11 12 and that this is not merely because of the enhanced expectation of improvement among participants in active treatment arms who experience side effects and guess their treatment allocation. 13 Around 25% of trial participants taking antidepressants experience a substantial effect, compared with around 10% taking placebos. 14
However, the review discusses an important point—that most of the public believes the chemical imbalance theory is established, 15 and this is probably because general practitioners use it to justify prescribing antidepressants, although the only evidence cited to support this assertion was a small online survey. 16 While most GPs surveyed acknowledged chemical imbalance as one possible cause of depression, they ranked it last among 13 biological, psychological, and social factors, suggesting they believed in a much broader overall model of depression. 16
Unfortunately, the chemical imbalance explanation may have encouraged long term use of SSRIs because it falsely implies a serotonin deficiency needing long term replacement, perhaps for life. This false belief was identified in 10 qualitative studies of barriers and facilitators to discontinuing antidepressants when appropriate. 17 SSRIs may cause side effects, including gastrointestinal bleeding and sexual dysfunction. 12 Long term use of antidepressants may make it more difficult to come off treatment 18 and is associated with an increased risk of serious adverse events in older adults. 19 Therefore we should not tell people with depression that antidepressants correct an imbalance or deficiency of serotonin, or that they will necessarily need long term treatment.
Open and honest discussion with patients about the remaining uncertainties is essential. We do not know why antidepressants work well for some people and not others, or why they cause harm to some people, not others. Research into their biological and psychosocial mechanisms of action must continue. Trial evidence makes clear that the effect of antidepressants is on average modest. 10 The National Institute for Health and Care Excellence (NICE) therefore recommends that psychological therapy should be offered first (if available) to people with a new episode of less severe depression unless they prefer antidepressant treatment, and that people with more severe depression are given a combination of antidepressant and psychological treatment. 12
NICE recommends that clinicians advise people taking antidepressants for a first episode to take them for at least six months after recovery. 12 Roughly half of patients treated for nine months or more may be able to taper off antidepressants without relapsing and needing to restart. 20 People needing treatment for a second episode of depression are at greater risk of relapse after discontinuation, particularly if symptoms persist that are serious enough to impair daily activities, or their depression has an ongoing underlying cause. They may be advised to continue antidepressants for two years before considering stopping treatment again. 12
Trust between the prescriber and the person with depression is of paramount importance for a good outcome. An initial time frame for treatment should therefore be agreed, with frequent contact until symptoms have receded. 12 Personal continuity of care should be offered at six monthly regular reviews of longer term treatment, to optimise knowledge of the person and their situation. 12
Competing interests: The BMJ has judged that there are no disqualifying financial ties to commercial companies. The authors declare the following other interests: TK declares that his employer, the University of Southampton, has received funding from the National Institute for Health Research for his research on the assessment and treatment of depression in primary care, and on testing internet and telephone support to people wanting to come off long term antidepressants. TK was a member of the NICE depression guideline committee. SC has a history of depression and long term antidepressant use.
Provenance and peer review: Commissioned; externally peer reviewed.
- Moncrieff J ,
- Cooper RE ,
- Stockmann T ,
- Amendola S ,
- Hengartner MP ,
- Horowitz MA
- ↵ Blanchard S. Depression is not caused by chemical imbalance in the brain, say experts—and pills “not based on science.” Sun 2022 Jul 21. https://www.thesun.co.uk/health/19254920/depression-chemical-imblance-pills-not-work/
- ↵ Ely J. Depression “is not caused by low serotonin levels”: study casts doubt over widespread use of potent drugs designed to treat chemical imbalance in brain. MailOnline 2022 Jul 20. https://www.dailymail.co.uk/health/article-11027847/Depression-NOT-caused-low-serotonin-levels-study.html
- ↵ Knibbs J. Depression not caused by low serotonin as new study debunks chemical imbalance theory Daily Mirror 2022 Jul 21. https://www.mirror.co.uk/lifestyle/health/depression-not-caused-low-serotonin-27538041
- ↵ Hayward E. Antidepressants study casts doubt on drugs taken by 8m people. Times 2022 Jul 20. https://www.thetimes.co.uk/article/antidepressants-study-casts-doubt-on-drugs-taken-by-8m-people-db2dsb9zf
- ↵ Science Media Centre. Expert reaction to a review paper on the “serotonin theory of depression.” 2022. https://www.sciencemediacentre.org/expert-reaction-to-a-review-paper-on-the-serotonin-theory-of-depression/
- ↵ Moncrieff J. Breakfast News, on the chemical imbalance myth. GB News, Jul 22. https://www.youtube.com/watch?v=2Ub7V0RcLew
- ↵ Moncrieff J, Horowitz M. Depression is probably not caused by a chemical imbalance in the brain—new study. The Conversation 2022 Jul 20. https://theconversation.com/depression-is-probably-not-caused-by-a-chemical-imbalance-in-the-brain-new-study-186672
- Cipriani A ,
- Furukawa TA ,
- Salanti G ,
- ↵ NICE. Depression in adults: treatment and management. 2022. www.nice.org.uk/guidance/ng222
- Hieronymus F ,
- Lisinski A ,
- Nilsson S ,
- Yaseen ZS ,
- Miller BJ ,
- Richardville K ,
- Kalaria SN ,
- Pilkington PD ,
- Reavley NJ ,
- Dewar-Haggart R ,
- Williams S ,
- Coupland C ,
- Morriss R ,
- Hippisley-Cox J
- Marston L ,
Psychological Theories of Depression
Saul Mcleod, PhD
Editor-in-Chief for Simply Psychology
BSc (Hons) Psychology, MRes, PhD, University of Manchester
Saul Mcleod, PhD., is a qualified psychology teacher with over 18 years of experience in further and higher education. He has been published in peer-reviewed journals, including the Journal of Clinical Psychology.
Learn about our Editorial Process
Olivia Guy-Evans, MSc
Associate Editor for Simply Psychology
BSc (Hons) Psychology, MSc Psychology of Education
Olivia Guy-Evans is a writer and associate editor for Simply Psychology. She has previously worked in healthcare and educational sectors.
On This Page:
Depression is a mood disorder that prevents individuals from leading a normal life at work, socially, or within their family. Seligman (1973) referred to depression as the ‘common cold’ of psychiatry because of its frequency of diagnosis.
Depending on how data are gathered and how diagnoses are made, as many as 27% of some population groups may be suffering from depression at any one time (NIMH, 2001; data for older adults).
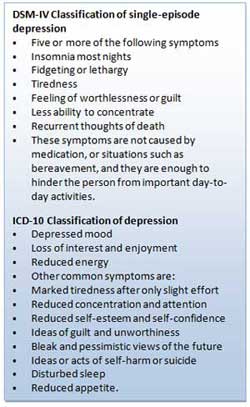
Behaviorist Theory
Behaviorism emphasizes the importance of the environment in shaping behavior. The focus is on observable behavior and the conditions through which individuals” learn behavior, namely classical conditioning, operant conditioning, and social learning theory.
Therefore, depression is the result of a person’s interaction with their environment.
For example, classical conditioning proposes depression is learned through associating certain stimuli with negative emotional states. Social learning theory states behavior is learned through observation, imitation, and reinforcement.
Operant Conditioning
Operant conditioning states that depression is caused by the removal of positive reinforcement from the environment (Lewinsohn, 1974). Certain events, such as losing your job, induce depression because they reduce positive reinforcement from others (e.g., being around people who like you).
Depressed people usually become much less socially active. In addition, depression can also be caused by inadvertent reinforcement of depressed behavior by others.
For example, when a loved one is lost, an important source of positive reinforcement has lost as well. This leads to inactivity. The main source of reinforcement is now the sympathy and attention of friends and relatives.
However, this tends to reinforce maladaptive behavior, i.e., weeping, complaining, and talking of suicide. This eventually alienates even close friends leading to even less reinforcement and increasing social isolation and unhappiness. In other words, depression is a vicious cycle in which the person is driven further and further down.
Also, if the person lacks social skills or has a very rigid personality structure, they may find it difficult to make the adjustments needed to look for new and alternative sources of reinforcement (Lewinsohn, 1974). So they get locked into a negative downward spiral.
Critical Evaluation
Behavioral/learning theories make sense in terms of reactive depression, where there is a clearly identifiable cause of depression. However, one of the biggest problems for the theory is that of endogenous depression. This is depression that has no apparent cause (i.e., nothing bad has happened to the person).
An additional problem of the behaviorist approach is that it fails to consider cognitions (thoughts) influence on mood.
Psychodynamic Theory
During the 1960s, psychodynamic theories dominated psychology and psychiatry. Depression was understood in terms of the following:
- inwardly directed anger (Freud, 1917),
- introjection of love object loss,
- severe super-ego demands (Freud, 1917),
- excessive narcissistic , oral, and/or anal personality needs (Chodoff, 1972),
- loss of self-esteem (Bibring, 1953; Fenichel, 1968), and
- deprivation in the mother-child relationship during the first year (Kleine, 1934).
Freud’s psychoanalytic theory is an example of the psychodynamic approach . Freud (1917) proposed that many cases of depression were due to biological factors.
However, Freud also argued that some cases of depression could be linked to loss or rejection by a parent. Depression is like grief in that it often occurs as a reaction to the loss of an important relationship.
However, there is an important difference because depressed people regard themselves as worthless. What happens is that the individual identifies with the lost person so that repressed anger towards the lost person is directed inwards towards the self. The inner-directed anger reduces the individual’s self-esteem and makes him/her vulnerable to experiencing depression in the future.
Freud distinguished between actual losses (e.g., the death of a loved one) and symbolic losses (e.g., the loss of a job). Both kinds of losses can produce depression by causing the individual to re-experience childhood episodes when they experience loss of affection from some significant person (e.g., a parent).
Later, Freud modified his theory stating that the tendency to internalize lost objects is normal and that depression is simply due to an excessively severe super-ego. Thus, the depressive phase occurs when the individual’s super-ego or conscience is dominant. In contrast, the manic phase occurs when the individual’s ego or rational mind asserts itself, and s/he feels control.
In order to avoid loss turning into depression, the individual needs to engage in a period of mourning work, during which s/he recalls memories of the lost one.
This allows the individual to separate himself/herself from the lost person and reduce inner-directed anger. However, individuals very dependent on others for their sense of self-esteem may be unable to do this and so remain extremely depressed.
Psychoanalytic theories of depression have had a profound impact on contemporary theories of depression.
For example, Beck’s (1983) model of depression was influenced by psychoanalytic ideas such as the loss of self-esteem (re: Beck’s negative view of self), object loss (re: the importance of loss events), external narcissistic deprivation (re: hypersensitivity to loss of social resources) and oral personality (re: sociotropic personality).
However, although highly influential, psychoanalytic theories are difficult to test scientifically. For example, its central features cannot be operationally defined with sufficient precision to allow empirical investigation. Mendelson (1990) concluded his review of psychoanalytic theories of depression by stating:
“A striking feature of the impressionistic pictures of depression painted by many writers is that they have the flavor of art rather than of science and may well represent profound personal intuitions as much as they depict they raw clinical data” (p. 31).
Another criticism concerns the psychanalytic emphasis on the unconscious, intrapsychic processes, and early childhood experience as being limiting in that they cause clinicians to overlook additional aspects of depression. For example, conscious negative self-verbalization (Beck, 1967) or ongoing distressing life events (Brown & Harris, 1978).
Cognitive Explanation of Depression
This approach focuses on people’s beliefs rather than their behavior. Depression results from systematic negative bias in thinking processes.
Emotional, behavioral (and possibly physical) symptoms result from cognitive abnormality. This means that depressed patients think differently from clinically normal people. The cognitive approach also assumes changes in thinking precede (i.e., come before) the onset of a depressed mood.
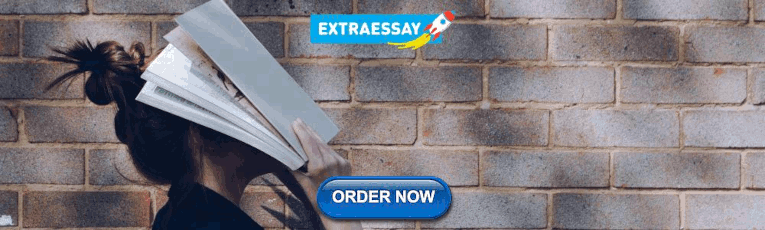
Beck’s (1967) Theory
One major cognitive theorist is Aaron Beck. He studied people suffering from depression and found that they appraised events in a negative way.
Beck (1967) identified three mechanisms that he thought were responsible for depression:
The cognitive triad (of negative automatic thinking) Negative self schemas Errors in Logic (i.e. faulty information processing)
The cognitive triad is three forms of negative (i.e., helpless and critical) thinking that are typical of individuals with depression: namely, negative thoughts about the self, the world, and the future. These thoughts tended to be automatic in depressed people as they occurred spontaneously.
For example, depressed individuals tend to view themselves as helpless, worthless, and inadequate. They interpret events in the world in an unrealistically negative and defeatist way, and they see the world as posing obstacles that can’t be handled.
Finally, they see the future as totally hopeless because their worthlessness will prevent their situation from improving.
As these three components interact, they interfere with normal cognitive processing, leading to impairments in perception, memory, and problem-solving, with the person becoming obsessed with negative thoughts.
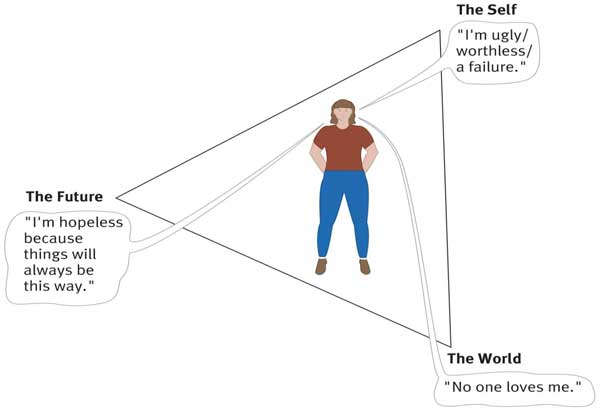
Beck believed that depression-prone individuals develop a negative self-schema . They possess a set of beliefs and expectations about themselves that are essentially negative and pessimistic. Beck claimed that negative schemas might be acquired in childhood as a result of a traumatic event. Experiences that might contribute to negative schemas include:
- Death of a parent or sibling.
- Parental rejection, criticism, overprotection, neglect, or abuse.
- Bullying at school or exclusion from a peer group.
However, a negative self-schema predisposes the individual to depression, and therefore someone who has acquired a cognitive triad will not necessarily develop depression.
Some kind of stressful life event is required to activate this negative schema later in life. Once the negative schema is activated, a number of illogical thoughts or cognitive biases seem to dominate thinking .
People with negative self-schemas become prone to making logical errors in their thinking, and they tend to focus selectively on certain aspects of a situation while ignoring equally relevant information.
Beck (1967) identified a number of systematic negative biases in information processing known as logical errors or faulty thinking. These illogical thought patterns are self-defeating and can cause great anxiety or depression for the individual. For example:
- Arbitrary Inference: Drawing a negative conclusion in the absence of supporting data.
- Selective Abstraction: Focusing on the worst aspects of any situation.
- Magnification and Minimisation: If they have a problem, they make it appear bigger than it is. If they have a solution they make it smaller.
- Personalization: Negative events are interpreted as their fault.
- Dichotomous Thinking: Everything is seen as black and white. There is no in between.
Such thoughts exacerbate and are exacerbated by the cognitive triad. Beck believed these thoughts or this way of thinking become automatic.
When a person’s stream of automatic thoughts is very negative, you would expect a person to become depressed. Quite often these negative thoughts will persist even in the face of contrary evidence.
Alloy et al. (1999) followed the thinking styles of young Americans in their early 20s for six years. Their thinking style was tested, and they were placed in either the ‘positive thinking group’ or ‘negative thinking group’.
After six years, the researchers found that only 1% of the positive group developed depression compared to 17% of the ‘negative’ group. These results indicate there may be a link between cognitive style and the development of depression.
However, such a study may suffer from demand characteristics. The results are also correlational. It is important to remember that the precise role of cognitive processes is yet to be determined. The maladaptive cognitions seen in depressed people may be a consequence rather than a cause of depression.
Learned Helplessness
Martin Seligman (1974) proposed a cognitive explanation of depression called learned helplessness .
According to Seligman’s learned helplessness theory, depression occurs when a person learns that their attempts to escape negative situations make no difference.
Consequently, they become passive and will endure aversive stimuli or environments even when escape is possible.
Seligman based his theory on research using dogs.
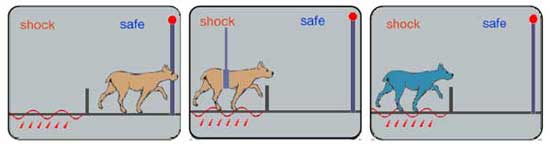
A dog put into a partitioned cage learns to escape when the floor is electrified. If the dog is restrained whilst being shocked, it eventually stops trying to escape.
Dogs subjected to inescapable electric shocks later failed to escape from shocks even when it was possible to do so. Moreover, they exhibited some of the symptoms of depression found in humans (lethargy, sluggishness, passive in the face of stress, and appetite loss).
This led Seligman (1974) to explain depression in humans in terms of learned helplessness , whereby the individual gives up trying to influence their environment because they have learned that they are helpless as a consequence of having no control over what happens to them.
Although Seligman’s account may explain depression to a certain extent, it fails to take into account cognitions (thoughts). Abramson, Seligman, and Teasdale (1978) consequently introduced a cognitive version of the theory by reformulating learned helplessness in terms of attributional processes (i.e., how people explain the cause of an event).
The depression attributional style is based on three dimensions, namely locus (whether the cause is internal – to do with a person themselves, or external – to do with some aspect of the situation), stability (whether the cause is stable and permanent or unstable and transient) and global or specific (whether the cause relates to the “whole” person or just some particular feature characteristic).
In this new version of the theory, the mere presence of a negative event was not considered sufficient to produce a helpless or depressive state. Instead, Abramson et al. argued that people who attribute failure to internal, stable, and global causes are more likely to become depressed than those who attribute failure to external, unstable, and specific causes.
This is because the former attributional style leads people to the conclusion that they are unable to change things for the better.
Gotlib and Colby (1987) found that people who were formerly depressed are actually no different from people who have never been depressed in terms of their tendencies to view negative events with an attitude of helpless resignation.
This suggests that helplessness could be a symptom rather than a cause of depression. Moreover, it may be that negative thinking generally is also an effect rather than a cause of depression.
Humanist Approach
Humanists believe that there are needs that are unique to the human species. According to Maslow (1962), the most important of these is the need for self-actualization (achieving our potential). The self-actualizing human being has a meaningful life. Anything that blocks our striving to fulfill this need can be a cause of depression. What could cause this?
- Parents impose conditions of worth on their children. I.e., rather than accepting the child for who s/he is and giving unconditional love , parents make love conditional on good behavior. E.g., a child may be blamed for not doing well at school, develop a negative self-image and feel depressed because of a failure to live up to parentally imposed standards.
- Some children may seek to avoid this by denying their true selves and projecting an image of the kind of person they want to be. This façade or false self is an effort to please others. However, the splitting off of the real self from the person you are pretending to cause hatred of the self. The person then comes to despise themselves for living a lie.
- As adults, self-actualization can be undermined by unhappy relationships and unfulfilling jobs. An empty shell marriage means the person is unable to give and receive love from their partner. An alienating job means the person is denied the opportunity to be creative at work.
Abramson, L. Y., Seligman, M. E., & Teasdale, J. D. (1978). Learned helplessness in humans: critique and reformulation . Journal of abnormal psychology, 87(1) , 49.
Alloy, L. B., Abramson, L. Y., Whitehouse, W. G., Hogan, M. E., Tashman, N. A., Steinberg, D. L., … & Donovan, P. (1999). Depressogenic cognitive styles : Predictive validity, information processing and personality characteristics, and developmental origins. behavior research and therapy, 37(6) , 503-531.
Beck, A. T. (1967). Depression: Causes and treatment . Philadelphia: University of Pennsylvania Press.
Beck, A. T., Epstein, N., & Harrison, R. (1983). Cognitions, attitudes and personality dimensions in depression. British Journal of Cognitive Psychotherapy .
Bibring, E. (1953). The mechanism of depression .
Brown, G. W., & Harris, T. (1978). Social origins of depression: a reply. Psychological Medicine, 8(04) , 577-588.
Chodoff, P. (1972). The depressive personality: A critical review. Archives of General Psychiatry, 27(5) , 666-673.
Fenichel, O. (1968). Depression and mania. The Meaning of Despair . New York: Science House.
Freud, S. (1917). Mourning and melancholia. Standard edition, 14(19) , 17.
Gotlib, I. H., & Colby, C. A. (1987). Treatment of depression: An interpersonal systems approach. Pergamon Press.
Klein, M. (1934). Psychogenesis of manic-depressive states: contributions to psychoanalysis . London: Hogarth.
Lewinsohn, P. M. (1974). A behavioral approach to depression .
Maslow, A. H. (1962). Towards a psychology of being . Princeton: D. Van Nostrand Company.
National Institute of Mental Health. (2001). Depression research at the National Institute of Mental Health http://www.nimh.nih.gov/health/publications/depression/complete-index.shtml.
Seligman, M. E. (1973). Fall into helplessness. Psychology today, 7(1) , 43-48.
Seligman, M. E. (1974). Depression and learned helplessness . John Wiley & Sons.
Further Information
- List of Support Groups
- Campaign against Living Miserably
- Men do cry: one man’s experience of depression
- NHS Self Help Guides

- Open access
- Published: 16 February 2021
On inflammatory hypothesis of depression: what is the role of IL-6 in the middle of the chaos?
- Elnaz Roohi 1 ,
- Nematollah Jaafari 2 &
- Farshad Hashemian 1
Journal of Neuroinflammation volume 18 , Article number: 45 ( 2021 ) Cite this article
13k Accesses
80 Citations
16 Altmetric
Metrics details
Many patients with major depressive disorder (MDD) are reported to have higher levels of multiple inflammatory cytokines including interleukin 6 (IL-6). Recent studies both pre-clinical and clinical have advocated for the functional role of IL-6 in development of MDD and suggested a great potential for targeting this cytokine to open new avenues in pharmacotherapy of depression. The purpose of the present narrative review was to provide an integrated account of how IL-6 may contribute to development of depression. All peer-reviewed journal articles published before July 2020 for each area discussed were searched by WOS, PubMed, MEDLINE, Scopus, Google Scholar, for original research, review articles, and book chapters. Publications between 1980 and July 2020 were included. Alterations in IL-6 levels, both within the periphery and the brain, most probably contribute to depression symptomatology in numerous ways. As IL-6 acts on multiple differing target tissues throughout the body, dysregulation of this particular cytokine can precipitate a multitude of events relevant to depression and blocking its effects can prevent further escalation of inflammatory responses, and potentially pave the way for opening new avenues in diagnosis, treatment, and prevention of this debilitating disorder.
Major depressive disorder (MDD) is a leading cause of disability throughout the world with a global prevalence of 2.6–5.9% [ 1 ]. The total estimated number of people living with depression worldwide increased by 49.86% from 1990 to 2017 [ 2 ]. According to worldwide projections, MDD will be the single major cause of burden of all health conditions by 2030 [ 3 ]. MDD is characterized by periods of low mood, altered cognition, considerable functional burden including impaired occupational functioning and psychosocial disability [ 4 ]. Despite available pharmacotherapeutic options, 30–60% of patients with MDD are not responsive to available treatments [ 5 ] and the rate of remission of the disease is often < 50% [ 6 ], while recurrence rates are more than 85% within 10 years of a depressive episode, and average about ≥ 50% within 6 months of assumed clinical remission [ 4 ]. Indeed, there exists no compelling evidence that current treatments are capable of disease modification in MDD patients. Thus, therapeutic deficiency in treatment outcomes reflects the demand for revitalizing psychiatric therapeutics with novel pharmacotherapeutic options that engage non-monoaminergic molecular targets.
A large body of evidence suggests that inflammation has central role in pathogenesis of MDD [ 7 , 8 , 9 , 10 , 11 , 12 , 13 ]. However, the exact mechanisms underlying inflammation-induced depression are not completely elucidated [ 3 ]. Historically, the “monoamine-depletion hypothesis” has been the main proposed pathophysiology [ 14 ]; nevertheless, this hypothesis alone cannot fully account for pathogenesis of MDD [ 15 , 16 ]. In recent years, “inflammatory hypothesis” has been proposed [ 17 ]. However, it is noteworthy that it was probably in the early 1990s that for the first time, possible relationships between the peripheral immune system and major depression was studied [ 18 ]. Maes et al. (1992) established immune cell profile of patients with depression and advocated for the existence of a systemic immune activation during major depressive disorder [ 19 ]. Moreover, correlations between IL-6 activity, acute phase proteins, and hyperactivity of the hypothalamic-pituitary-adrenal (HPA) axis were suggested in severe depression [ 20 ].
Most proximally, inflammation is regulated by expression of immune response genes including interleukin (IL)-1B, tumor necrosis factor (TNF), and IL-6 which promote secretion of pro-inflammatory cytokines leading to systemic inflammation. Distally, inflammation is regulated in the brain where socio-environmental cues including possible threat are detected. This neuro-inflammatory link can activate the conserved transcriptional response to adversity (CTRA) before happening of a possible threat or bacterial infection. However, the negative aspect of central regulation of systemic inflammation is that it can give social and foreseen dangers (including those that have not yet occurred or may never actually happen) the ability to activate the CTRA in the absence of actual physical danger. Under normal conditions, CTRA-related inflammatory activity is downregulated by the HPA axis via the production of cortisol. Nevertheless, when prolonged actual or perceived social threat or physical danger is present, glucocorticoid resistance can develop which leads to excessive inflammation that heightens a person’s risk for development of several disorders including MDD, especially if activation of these pathways is prolonged [ 21 ]. As mentioned above, the current understanding of MDD encloses not only alterations in neurotransmitters, but also changes in immune and endocrine functioning as well as neural circuits [ 22 ]. This broadened framework has just started to inform a wide array of novel, personalized therapeutics that are showcasing great promise in a new holistic approach to MDD [ 23 ].
Cytokines are implicated in pathogenesis of MDD [ 24 , 25 , 26 , 27 , 28 , 29 , 30 ]. Risk factors of developing MDD include familial, developmental, psychological, and medical risk factors as well as molecular factors associated with genetics, epigenetics, gene expression, and also those related to the endocrine and the immune system [ 31 , 32 ]. All these risk factors have been shown to be related with changes in cytokine production or signaling. In other words, cytokines are involved in almost every predisposing or precipitating risk factor associated with MDD [ 24 ]. Indeed, there is accumulating evidence in favor of involvement of pro-inflammatory cytokines in pathophysiology of depression [ 24 , 29 , 33 , 34 , 35 , 36 ]. Various studies reported higher levels of multiple inflammatory markers including IL-6 in patients with MDD [ 37 , 38 , 39 , 40 , 41 ]. Of all pro-inflammatory cytokines, changes in IL-6 serum levels have been reported as one of the most reproducible abnormalities in MDD [ 38 ].
The aim of the present narrative review is to elucidate the fundamentals, implications, challenges of cytokine research specifically IL-6 in major depressive disorder. This comprises of the following:
-) A Brief overview of cytokines
-) Cytokine categories according to immunological function.
-) IL-6 as a pleiotropic cytokine.
-) Brief overview of chemokines and their role in Depression.
-) Challenges of cytokine research in psychiatry.
-) IL-6 alterations in depression.
-) Effects of IL-6 on neurotransmitters’ synthesis, signaling, metabolism, and function.
-) Effects of IL-6 levels on brain morphology in depression.
-) Blockade of IL-6 and its receptor in the periphery as a potential therapeutic option in MDD.
-) Possible role of IL-6 together with gut microbiota in pathogenesis of depression.
-) Elevated levels of IL-6 in patients with COVID-19 infection.
The present article is a narrative review. All peer-reviewed journal articles published before July 2020 for each area discussed were searched by WOS, PubMed, MEDLINE, Scopus, Google Scholar, for original research, review articles, and book chapters. We selected articles on the basis of being comprehensive, innovative, and informative for an in-depth understanding and a critical debate on the topic. Publications between 1973 and 2020 were included.
A brief overview of cytokines
Cytokines are a broad category of released proteins that act as signaling molecules to regulate inflammation and cellular activities [ 24 , 42 ]. They are produced by different immune cells (e.g., macrophages, lymphocytes, mast cells), parenchymal cells, endothelial and epithelial cells, fibroblasts, adipocytes, and stromal cells within the periphery [ 24 , 43 ]. Additionally, they are produced by microglia, astrocytes, and neurons in the brain [ 44 ]. Cytokines from the periphery (peripherally produced cytokines) can exert influences on inflammatory processes in the brain [ 45 , 46 ]. Indeed, they can enter blood-brain barrier (BBB) and affect the brain via humoral (accessing the brain through leaky secretions of the BBB such as choroid plexus), neural (through stimulation of primary afferent nerve fibers in the vagus nerve), and cellular (through stimulation of microglia by pre-inflammatory cytokines to produce monocyte chemottractant protein-1 and recruit monocytes to the brain) pathways [ 47 ]. Most cytokines function in their immediate microenvironment. Few of them are involved in paracrine signaling which indeed is fundamental to the control of an inflammatory response within a given tissue or organ and the activation of a coordinated immune response that involves multiple cell types [ 48 ]. Apart from navigating the immune system to defend the body from pathogens, cytokines have a modifying effect on neurotransmission [ 49 ].
It’s also noteworthy that the same cytokines can be produced by multiple cell types. For example, white blood cells, endothelium, fat cells, and other cells can produce TNF-α [ 50 ]. Additionally, one single cell can release different cytokines. For instance, T Helper type 2 (T H 2) cells can produce IL-3, IL-4, IL-5, IL-6, and IL-13 [ 24 ]. Cytokines can have pleiotropic, redundant, synergistic, and antagonistic effects [ 51 ]. The phenomenon that a single cytokine can act on several different cell types is called pleiotropy [ 51 ]. For instance, IL-10 can activate T H 2 cells and B cells, yet inhibit macrophages and T helper type 1 (T H 1) cells. Thus, being immunostimulatory as well as being immunosuppressive [ 52 ]. Cytokines are redundant in their activity, i.e., similar functions can be exerted by different cytokines. For instance, interferon (IFN)-γ, IL-2, and TNF-α enhance cellular immunity and production of cytotoxic cell contacts [ 53 ]. Cytokines can also act synergistically, i.e., they can have combined effects when acting together. For instance, IL-3 and IL-4 amplify each other’s effects to induce growth, differentiation, and activation of mast cells in a synergistic manner [ 24 ]. Another phenomenon in cytokines signaling is antagonism. An example of cytokine antagonism is that cytokines of the IL-1 superfamily can antagonize IL-18 effects [ 54 ].
Cytokine categories according to immunological function
Four categories of cytokines are usually referred to in psychoimmunological literature. (1) T H 1 cytokines (IL-2, IL-12, IFN-γ) which induce cytotoxic cell contacts. (2) T H 2 cytokines (IL-4, (IL-5, IL-13) which lead to production of antibodies. (3) Pro-inflammatory cytokines (IL-1, IL-6, IL-8, IL-17, IL-21, IL-22, IFN-α, TNF-α) which further the progress of inflammation. (4) Anti-inflammatory cytokines (IL-10, transforming growth factor-beta (TGF)-β which are influenced by regulatory T cells and impede inflammatory process from escalating [ 24 ]. However, these categories are not distinct and it must be considered that cytokines can exert various effects on different cells and therefore, they may have pro- and also anti-inflammatory properties. For instance, IFN-α which has been listed as a pro-inflammatory cytokine can also have anti-inflammatory properties [ 55 ].
IL-6 as a pleiotropic cytokine
IL-6 was first identified as a differentiation factor for B cells which stimulates production of antibodies by activated B cells. Apart from regulation of acute inflammation, IL-6 is known to induce differentiation of B cells, and activation and population expansion of T cells [ 56 ]. Within the peripheral and central nervous system (CNS), IL-6 can act as a neuronal growth factor inducing neurite development and nerve regeneration [ 57 ]. IL-6 receptor (IL-6R) consists of the IL-6-binding chain which has two forms of transmembrane IL-6R and soluble IL-6R (sIL-6R) [ 58 ] and a gp130 signal-transducing chain [ 59 ]. Following binding to its receptor (IL-6R), IL-6 initiates to exert its multiple functions.
It is quite interesting that IL-6 exerts both pro- and anti-inflammatory properties [ 60 , 61 ]. Indeed, its signaling is complex and can lead to both inflammatory and anti-inflammatory cascades depending upon the presence of either IL-6 receptor (IL-6R) or the membrane bound gp130 signal transducer and these are expressed at very different frequencies within specific cell type in the body [ 5 ]. Trans-signaling of IL-6, in which the soluble form of the IL-6 receptor (sIL-6R) is shed from the membrane bound receptors, is known to be pro-inflammatory [ 62 ]. The sIL-6R binds to IL-6 and is transported to any cell type on which gp130 is expressed [ 63 ]. While most soluble receptors (e.g., soluble receptor for TNFα) result in antagonistic action by competing for the ligand, the sIL-6R is agonistic and increases the types of cells through which IL-6 can signal. Additionally, IL-6 engages in classical signaling which is anti-inflammatory [ 63 ] and occurs through binding of IL-6 to the membrane bound cell surface receptor. Classical signaling of IL-6 solely occurs on some subsets of T cells, neutrophils and monocytes megakaryocytes, and hepatocytes [ 64 ]. In both classical and trans-signaling, the IL-6/IL-6R/gp130 complex uses two pathways to activate intracellular signaling namely the Janus kinase/signal transducer and activator of transcription (JAK/STAT) pathway and the mitogen-activated protein kinase (MAPK) pathway [ 5 ].
Indeed, IL-6 has been mostly regarded as having pro-inflammatory properties; however, it has many anti-inflammatory functions which are necessary for resolution of inflammation [ 65 ]. For instance, IL-6 inhibits activity of the transcription factor named nuclear factor kappa-light-chain-enhancer of activated B cells (NF-κB) and expression of the chemokine receptor on dendritic cells which is needed for recruiting these cells to lymphoid tissues; thus, involving in resolution of inflammation [ 66 ]. Research findings showed that IL-6 has a crucial role in regulation of T helper17 (Th17)/regulatory T (Treg) cells [ 67 ]. In the presence of TGF-β, IL-6 is a vital signal for differentiation of naive T cells into Th17 cells which in turn are implicated in induction of autoimmune diseases [ 68 , 69 ], and result in local tissue injury in chronic inflammatory disorders [ 70 ]. On the contrary, IL-6 can strongly inhibit the TGF-β-induced differentiation of Treg cells which in turn results in inhibition of autoimmunity and protects against tissue damage [ 71 ]. Functional dichotomy of IL-6 indicates that it may be responsible for maintaining the balance between pro- and anti-inflammatory responses, while having tissue-specific properties at the periphery and in the CNS [ 72 ].
Brief overview of chemokines and their role in depression
Chemokines are small chemotactic cytokines that are identified to have significant roles in migration of immune cells, induction of direct chemotaxis, and propagation of inflammatory responses [ 73 ]. They are classified into four sub-families based on their structural criteria (i.e., the number and spacing of their two N-terminals, disulfide bonding participating cysteine residues). These four subfamilies include CXC, CC, C, and CX3C [ 74 ]. Furthermore, they can be categorized according to their biological activity, namely, the maintenance of homeostasis and the induction of inflammation. There are also chemokines which have dual functionality [ 75 ].
These small chemotactic cytokines are known to be secreted in response to inflammatory cytokines; thereafter, selectively recruiting lymphocytes, monocytes, and neutrophil-inducing chemotaxis by activating G-protein-coupled receptors (GPCRs) [ 76 ]. A growing body of evidence suggests that chemotactic cytokines are implicated in neurobiological processes relevant to psychiatric disorders such as synaptic transmission and plasticity, neuroglia communication, and neurogenesis [ 77 ]. Indeed, disruption of any of the mentioned functions which may take place by activation of the inflammatory response system has consistently been found to be relevant in pathogenesis of depression [ 73 ].
There are indeed both pre-clinical and clinical evidence in support of linking alterations in the chemokine network to depressive behavior [ 73 ]. In an animal model of depression, namely prenatal stressed rats, levels of CCL2, and CXCL12 chemokines were found to be upregulated in the hippocampus and prefrontal cortex, which was indeed suggestive of excessive microglial activation [ 78 ]. Additionally, Trojan et al. (2017) investigated the modulatory properties of chronic administration of anti-depressants on the chemokines. According to their results, chronic administration of anti-depressants has been shown to normalize the prenatal stress-induced behavioral disturbances together with the observed alterations in CXCL12 and their receptor. Indeed, they concluded that alterations of CXCL12 and their receptor and at less extend changes in CX3CL1–CX3CR1 expression will probably be normalized following chronic treatment with anti-depressants [ 79 ].
Moreover, several clinical studies found correlations between elevated levels of circulating inflammatory chemokines and depressive symptoms in patients with major depressive disorder [ 73 , 80 , 81 , 82 , 83 ]. According to the results of a comprehensive meta-analysis, peripheral concentrations of a number of chemokines including CCL2, CCL3, CCL4, CCL11, CXCL4, CXCL7, and CXCL8 can potentially discriminate between individuals with depression and those without [ 84 ]. Additionally, Ślusarczyk et al. (2016) provided a comprehensive account of the role of chemokines in processes underlying depressive disorder [ 85 ].
Challenges of cytokine research in psychiatry
There are some difficulties faced by researchers in conducting cytokine research in psychiatry. The major problem seems to be heterogeneity of the obtained results. In other words, research outcomes are conflicting and challenging to interpret [ 24 ]. Moreover, research in this area is largely based on measurement of cytokine levels in the periphery and it is not completely clear how serum or plasma levels of cytokines reflect the situation in the brain [ 47 ]. Compellingly, results of studies that examined both peripheral and CFS levels of IL-6 found no correlations between the mentioned measures; thus, suggesting that peripheral levels of IL-6 may not directly reflect central IL-6 levels [ 86 , 87 ].
It is also noteworthy that some environmental, social, biological, and medical factors may influence peripheral cytokine changes. For instance, one of the characteristics of obesity is chronic inflammation with the increased circulating levels of cytokines [ 88 , 89 ]. Indeed, adipose tissue is reported to build up and activate lymphocytes and macrophages that secrete inflammatory factors [ 89 , 90 ]. Interestingly, obese people show behavioral symptoms such as MDD and cognitive dysfunction at an increased rate in comparison with the general population [ 91 , 92 ]. Therefore, one may argue that alterations in cytokine levels are somehow unspecific [ 24 ]. Additionally, there is a considerable overlap in cytokine values between patients in the acute phase of depression, patients in remission, and patients who are recovered [ 24 ]. Although the use of cytokines as potential biomarkers of depression has been discussed frequently in various studies, cytokine changes have been reported in other psychiatric disorders as well. For instance, increased levels of pro-inflammatory cytokines have been reported in generalized anxiety disorder [ 93 , 94 , 95 ], obsessive-compulsive disorder [ 96 ], posttraumatic stress disorder [ 97 , 98 ], and sleep disorder [ 99 ].
Moreover, cytokine levels change during pharmacotherapy of depression. Indeed, it has been suggested that treatment with anti-depressants can potentially lead to alteration in peripheral levels of cytokines. According to the results of a meta-analysis, anti-depressants, overall, cause decrease in peripheral levels of IL-6, IL-10, and TNF-α [ 100 ]. However, anti-psychotics which are used in psychotic depression, especially those with the highest risk of weight gain (e.g., clozapine and olanzapine), cause significant increase in the blood levels of pro-inflammatory cytokine [ 101 ]. Additionally, mood stabilizers such as lithium and carbamazepine have also been linked with an increase in the peripheral levels of cytokines [ 102 ]. In sum, as cytokine signaling often exhibits pleiotropic, redundant, synergistic, and antagonistic effects, it seems to be advisable to consider all cytokines that work together or against each other and therefore, take into account the whole range of cytokines instead of a single one.
On the role of interleukin-6 in depression
Il-6 alterations in depression.
A growing body of evidence suggests that IL-6 has a crucial role in pathogenesis of depression [ 3 ] and is the most consistently increased cytokine in blood samples of MDD patients [ 38 , 103 ]. The first promising evidence for the role of IL-6 in occurrence of depression is most probably provided by a longitudinal study in which children with higher circulating levels of IL-6 at age 9 were found to be at a 10% greater risk of developing MDD by age 18, compared to the general population or children with low IL-6 levels. Indeed, the researchers concluded that inflammation and high IL-6 levels possibly predate the occurrence of depression [ 104 ]. Another evidence for potential role of IL-6 in depression is that peripheral levels of IL-6 were found to be positively correlated with symptom severity in anti-depressant non-responders [ 105 ].
Stress-based preclinical models of depression showed that IL-6 levels are increased following the onset of depression-associated behaviors. Rodents who were exposed to chronic mild stress exhibited anhedonia and elevated circulating levels of pro-inflammatory cytokines including IL-6 [ 106 , 107 ]. Moreover, in another study on male Wistar rats, serum levels of pro-inflammatory cytokines including IL-6 were reported to be higher in acute and restraint stress compared to non-stressed rats [ 108 ]. It is also noteworthy that some studies reported no significant alteration in peripheral levels of IL-6 in chronic mild stress models of depression [ 109 ]. Nevertheless, they reported elevated CNS levels of other inflammatory markers which probably reflects a time-dependent shift from peripheral to central cytokine activation or potential transport of the peripheral cytokines into CNS [ 109 ]. Another promising evidence was provided by studies on IL-6 knockout mice. Indeed, they were reported to be resistant to the development of depression-like phenotype following long-term light deprivation in the constant darkness, proposing a functional role for IL-6 in stress susceptibility [ 110 ]. Moreover, Ślusarczyk et al. (2015) found evidence for the role of prenatal stress as a priming factor that could exhibit effects on microglial cells and consequently lead to depressive-like disturbances in adult rat offspring. According to their results, the release of pro-inflammatory cytokines including IL-6 is enhanced in microglia obtained from prenatally stressed animals compared to control animals [ 78 ].
In fact, not every individual exposed to prolonged or acute stress develops a psychiatric disorder [ 111 ]. According to previous research, vulnerability to repeated social defeat stress is predicted by differences in IL-6 levels in the innate peripheral immune system [ 112 ]. Following induction of social defeat stress, two thirds of mice were reported to show depression-like behavior measured by social avoidance, anhedonia, circadian system disruptions, and metabolic changes [ 113 ] together with elevated activation of pro-inflammatory cytokines such as IL-6 [ 112 ]. Indeed, higher degrees of elevation in peripheral IL-6 levels of susceptible mice were reported in comparison with resilient mice. Moreover, it was found that this increase occurs within 20 min of the first social defeat. Interestingly, mice that later became susceptible had higher number of leukocytes and those leukocytes produced more levels of IL-6 following stimulation via LPS ex vivo [ 112 ]. Additionally, studies with non-social stress-based models found evidence for the functional role of IL-6 in the development of stress susceptibility. In these models, animals were exposed to a controllable or uncontrollable stress (e.g., shock), and their ability to actively escape a subsequent stressor was measured. According to the results, 20% of animals who were exposed to uncontrollable stress were found susceptible and developed learned helplessness and the rest were found to be resilient. Interestingly, susceptible animals showed elevated levels of peripheral IL-6 together with anhedonia in contrast to resilient animals [ 114 ].
Clinical studies have also revealed that patients with MDD have increased levels of plasma and serum concentrations of pro-inflammatory cytokines including IL-6 in comparison with healthy controls [ 24 , 100 , 115 , 116 ]. It should be noted that three meta-analyses verified increased peripheral IL-6 levels in MDD patients compared to healthy volunteers [ 38 , 116 , 117 ]. Nevertheless, there are also studies reporting no significant differences in IL-6 levels in MDD patients compared to healthy volunteers [ 118 ]. However, one may argue that different subtypes of depression and certain depressive symptoms should be taken into account. For instance, Rudolf et al. (2014) compared IL-6 levels among patients with atypical and typical depression and healthy controls. According to their results, IL-6 levels were significantly increased in patients with atypical depression and not in typical MDD patients compared to healthy controls [ 119 ]. Additionally, Rush et al. (2016) studied peripheral levels of IL-6 and TGF-β in 55 melancholic depressed patients. They were found to have significantly higher baseline IL-6 levels compared to healthy controls. Moreover, these elevated levels of IL-6 did not normalize following electroconvulsive therapy (ECT) [ 120 ]. A recent systematic review conformed Rush et al.’s results. In the mentioned review, authors found that peripheral IL-6 levels are increased in patients with melancholic depression in comparison with controls [ 121 ]. Moreover, Maes et al. (1997) examined serum levels of IL-6 and IL-1 receptor antagonist in patients with chronic, treatment resistant depression both before and after subchronic treatment with anti-depressants. According to their results, subchronic treatment with anti-depressants had no significant impact on serum levels of IL-6; nevertheless, it decreased serum soluble IL-6R levels significantly [ 122 ].
Effects of IL-6 on neurotransmitters’ synthesis, signaling, metabolism, and function
The effects of cytokines on neurotransmitters have been studied extensively [ 49 , 123 ]. Cytokines and their signaling pathways (e.g., p38 mitogen activated protein kinase) are reported to exhibit significant impacts on metabolism of multiple neurotransmitters such as serotonin, dopamine, and glutamate; thus, influencing their synthesis, release, and reuptake [ 49 ]. Indeed, cytokines can decrease synthesis of serotonin via activating the enzyme indoleamine 2,3 dioxygenase (IDO) which breaks the precursor of serotonin (i.e., tryptophan) to kynurenine (KYN) instead of metabolizing tryptophan to serotonin; thus, leading to serotonin depletion [ 3 ]. The process of serotonin depletion has been long associated with major depression [ 124 , 125 ]. Moreover, cytokines can modulate serotonin signaling via elevating the expression and function of monoamine transporters. These transporters are known to re-uptake serotonin [ 126 , 127 ].
IL-6 is known to influence neurotransmission by modulating the behavioral output of the brain; however, the exact mechanism is unknown. A previous study showed that IL-6 directly controls the levels of serotonin transporter (SERT) and therefore influences serotonin reuptake. Indeed, the researchers concluded that IL6-induced modulation of serotonergic neurotransmission through the signal transducer and activator of transcription 3 (STAT3) signaling pathway contributes to the role of IL6 in depression [ 128 ]. The activity of SERT forms serotonergic transmission which is implicated in depressive behavioral changes and pathophysiology of the disease [ 129 ]. By intensifying dopaminergic and serotonergic turnover in hippocampus and frontal cortex, IL-6 influences neurotransmission of catecholamines [ 130 ]. It seems that noradrenaline is not affected by IL-6; however, noradrenaline itself can induce expression of IL-6 in glial cells [ 131 ]. IL-6 together with other pro-inflammatory cytokines can activate kinurenine pathway which is involved in glutamatergic neurotransmission [ 132 ].
Effects of IL-6 levels on brain morphology in depression
Previous studies showed that elevated levels of pro-inflammatory cytokines such as IL-6 may affect neurogenesis [ 133 ] and neural plasticity [ 134 ]. Imaging studies have shown that specific brain regions such as basal ganglia (which is involved in motor activity and motivation), the dorsal anterior cingulate cortex (ACC) (which has a central role in generation of anxiety), and the subgenual ACC (which is known to be involved in the development of depression) are influenced by cytokines [ 135 , 136 ]. Additionally, high IL-6 expression levels demonstrated neuropathologic manifestations including neurodegeneration [ 137 , 138 ].
There are many studies in which positron emission tomography (PET) has been applied to test translocator protein (TSPO) binding, a marker of neuroinflammation, in order to study neuroinflammatory hypothesis of depression [ 139 , 140 , 141 , 142 , 143 ]. According to their results, neuroinflammation was present in various regions of the brain (e.g., neocortical grey matter, frontal cortex, prefrontal cortex, anterior cingulate cortex, insula, temporal cortex) as well as the hippocampus [ 139 , 140 , 141 , 142 , 143 ].
In a recent study, Kakeda et al. (2018) evaluated possible relationship between serum levels of IL-1β, IL-6, IFN-γ, and TNFα and brain morphology in terms of brain cortical thinning and hippocampal subfield volumes during the first depressive episode in drug-naïve patients with MDD using a whole-brain SBM analysis. They found a significant inverse correlation between prefrontal cortex (PFC) thickness and serum IL-6 level in MDD patients. Indeed, high serum levels of IL-6 were correlated with reduced left subiculum and right CA1, CA3, CA4, GC-DG, subiculum, and whole hippocampus volumes in MDD patients. Additionally, thickness of the superior frontal and medial orbitofrontal cortices in patients with depression was significantly decreased compared to healthy controls. Since PFC contains high concentrations of IL-6 receptors, IL-6 mediated neurotoxicity might happen under conditions in which high serum IL-6 levels are present (i.e., early stages of MDD). Consequently, the authors advocated that the neuroinflammatory status in the early stage of MDD is associated with changes in the brain gray matter and IL-6 probably plays a key role in the morphological changes observed in the PFC during early stages of the disease. It is also noteworthy that serum IL-6 was the only cytokine among the tested cytokines that showed significant differences between the patients and controls in their study. Indeed, serum IL-6 levels were found to be significantly higher in MDD patients than in healthy controls [ 144 ]. In another study, Frodl et al. (2012) investigated possible effects of changes in the glucocorticoid and inflammatory systems on hippocampal volumes in patients with MDD. According to their results, MDD patients showed increased IL-6 levels and smaller hippocampal volumes compared to healthy controls. Positive effects of messenger RNA (mRNA) expression of glucocorticoid-inducible genes and further inverse effects of IL-6 concentration, on hippocampal volumes were also reported. Thus, they concluded that increased expression of IL-6 can probably predict decreased hippocampal volume [ 145 ].
As already mentioned, there is considerable amount of evidence regarding the central role of the highly plastic, stress-sensitive hippocampal region in pathogenesis of depression [ 146 ]. Indeed, grey-matter structures, including the hippocampus are vulnerable to atrophy in depression [ 147 , 148 ]. Hippocampal volume reductions are most probably the result of remodeling of key cellular elements, involving retraction of dendrites, loss of glial cells, and decreased neurogenesis in the dentate gyros [ 149 ]. Factors underlying this cellular remodeling are known to be stress-induced increased levels of glucocorticoids, which are implicated in decreased neurogenesis [ 150 ]. Moreover, increased activity of the HPA axis resulting in decreased levels of glucocorticoids combined with resistance to glucocorticoid-induced negative feedback control is commonly observed in depression [ 151 ]. This dysregulation of glucocorticoid secretion along with the increased activity of excitatory neurotransmitters can potentially lead to cellular remodeling (which can be reversible) and hippocampal neurons cell death in patients with depression [ 152 ]. Since hippocampus has been identified to have a role in negative feedback inhibition of glucocorticoids, remodeling or neuronal damage may lead to less efficient inhibitory control of the corticotrophin-releasing hormone, resulting in elevated amounts of circulating glucocorticoids and further damage of the hippocampal neurons [ 153 ]. Taken together, it seems that further studies are required to elucidate the physiological mechanisms in which IL-6 might exert changes in the brain grey matter. A brief overview of the effects of cytokines including IL-6 on brain morphology is shown in Fig. 1 .
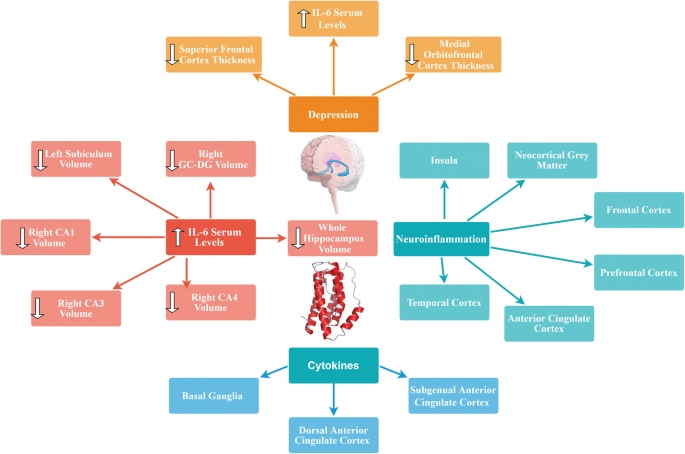
Effects of cytokines including IL-6 on brain morphology
Blockade of IL-6 and its receptor in the periphery as a potential therapeutic option in MDD
Growing body of evidence suggests that abnormalities in the immune system are most probably relevant to pathogenesis and potential novel treatment of psychiatric disorders. Previous studies showed that alterations in the peripheral IL-6 levels might contribute to depressive-like behavior in animal studies [ 3 , 112 , 114 , 154 ]. Moreover, IL-6 knockout mice showed resistance to development of depressive-like behavior [ 155 ] which gives further evidence for possible role of IL-6 pathogenesis of depression. High peripheral levels of IL-6 are even more apparent in patients with treatment-resistant depression. Additionally, correlations have been found between decrease in IL-6 levels and alleviation of depressive symptoms in patients who were responsive to the pharmacotherapy [ 156 ]. Moreover, results of a study on 222 stroke patients indicated significant associations between IL-6 periphery levels and development of MDD within 2 weeks and at 1 year following stroke. Furthermore, significant correlations were found between statin use and IL-6 on the presence of a depressive disorder at the 1st year. Indeed, preventive effects of treatment with statins (which are known to possess anti-inflammatory properties and potently reduce the cytokine-mediated IL-6 release [ 157 ]) against post-stroke depression was confirmed [ 158 ]. Thus, suppression of IL-6 activity could possibly lead to clinical recovery and may be considered as a novel pharmacotherapeutic option. Utilizing IL-6 receptor antibodies (for instance, Tocilizumab) or IL-6 antibodies (for instance, Sirukumab or Siltuximab) for reduction of IL-6 activity seems to be a novel strategy.
Blockade of IL-6 receptor by the humanized anti-IL-6 antibody, Tocilizumab has been used in treatment of rheumatoid arthritis (RA) [ 159 , 160 , 161 , 162 , 163 ] and systemic juvenile idiopathic arthritis [ 164 , 165 , 166 ]. Extensive clinical studies have established both short-term and long-term efficacy and safety of Tocilizumab [±conventional disease-modifying anti-rheumatic drugs (DMARDs)] in adults with moderate to severe RA. Additionally, Tocilizumab was shown to be effective as a monotherapy in patients with systemic juvenile idiopathic arthritis and also in patients whose disease has been refractory to other therapies [ 164 ]. Moreover, the safety profile of tocilizumab was reported to be consistent over time and also consistent with safety profile of other immunomodulatory agents [ 162 ]. It is also important to note that oral tocilizumab has been shown to inhibit experimental autoimmune encephalitis by elevating Th2 anti-inflammatory cytokines and decreasing pro-inflammatory Th1 cytokines [ 167 ]. With regard to crucial role of IL-6 in regulating metabolic homeostasis, side effects such as significant weight gain followed by hypertrygliceridemia and hypercholesterolemia may be observed in patients treated with tocilizumab [ 168 ]. Blockade of IL-6 trans-signaling, while classical IL-6R signaling stays intact seems to be crucial for the goal of maintaining gut mucosal integrity and epithelial regeneration [ 65 ]. Indeed, few randomized clinical trials were conducted on anti-depressant properties of tocilizumab. According to the results of a recent meta-analysis of anti-depressant activity of anti-cytokine therapies, treatment with tocilizumab showed statistically significant improvements in depressive symptoms [ 169 ].
Another promising human monoclonal antibody against Il-6, namely Sirukumab has been reported to be a safe and well-tolerated agent, capable of modulating the immune response in healthy populations as well as in patients with inflammatory disorders (e.g., rheumatoid arthritis). It targets the IL-6 signaling pathway by inhibition of both the pro- and anti-inflammatory effects of IL-6 [ 170 ]. Effects of Sirukumab on cytokine networks provide a well-founded rationale for its potential use in pharmacotherapy of psychiatric disorders promising possible advantages across varying domains of the biobehavioral research criteria [ 171 ]. In a phase 2, double-blind, placebo-controlled trial, the efficacy of Sirukumab and Siltuximab on depressive symptoms was studied in patients with rheumatoid arthritis or multicentric Castleman’s disease respectively. Compared with placebo, both IL-6 neutralizing antibodies were found to make significantly greater improvements on depressive symptoms in the patients [ 172 ]. Results of a recent mega-analysis of 18 randomized, placebo-controlled clinical trials of efficacy of immunomodulatory drugs on depressive symptoms in patients with inflammatory disorders demonstrated promising results ( N = 10,743 participants). According to their findings, anti-IL-6 antibodies (sirukumab and siltuximab) had large and statistically significant effect sizes on core depressive symptoms before correction for physical health outcomes. Additionally, their effects remained significant in non-responders for the primary disease states evaluated [ 173 ]. Although further research is needed in this area, potential application of anti-IL-6 antibodies could possibly open new avenues in pharmacotherapy of MDD.
Possible role of IL-6 together with gut microbiota in pathogenesis of depression
The human intestine harbors nearly 100 trillion bacteria [ 174 ] consisting assemblages of microorganisms associated with various niches in and on the body with long-term implications to health [ 175 ]. Evidence is emerging regarding correlations of microbial activities with progressive structural and functional processes in the brain of both animal models and humans [ 175 ]. There is a large body of evidence for the role of gut microbiota composition in pathogenesis of depression [ 176 , 177 , 178 , 179 , 180 ]. Moreover, there is growing body of literature for the influence of the gut microbiome on cytokine signaling [ 181 , 182 ].
The dominant gut microbial phyla are known to be Firmicutes and Bacteroideteds [ 183 , 184 ]. The Firmicutes / Bacteroideteds ratio is of great relevance in signaling human gut microbiota status [ 185 ]. For instance, increased levels of Firmicutes / Bacteroideteds ratio have been reported in patients suffering from irritable bowel syndrome (IBD) and seem to have some correlations with development of depression and anxiety [ 186 , 187 ]. Additionally, Firmicutes / Bacteroideteds ratio is associated with overall alterations in bacterial profiles at different life stages [ 185 ]. In a novel study, researchers reported decreased Firmicutes / Bacteroideteds ratio in mice following social defeat stress; thus, proposing possible role of Firmicutes / Bacteroideteds in depressive-like behavior. Furthermore, administration of anti-mouse IL-6 receptor antibody (MR16-1) attenuated the decreased ratio of Firmicutes / Bacteroideteds in susceptible mice. Thus, the researchers concluded that anti-mouse IL-6 receptor antibody may have anti-depressant-like effects by normalizing the Firmicutes / Bacteroideteds ratio via modulation of the immune system [ 3 ].
Decreased number of Oscillospira was detected in patients with depression [ 180 ] which suggests for possible role of Oscillospira in pathogenesis of depression. Two animal studies yielded same results. A recent study investigated therapeutic effects of finasteride on depressive-like behavior in rats together with 1 month of treatment withdrawal. Withdrawal from finasteride was associated with increased depressive-like behavioral responses. Therapeutic use of finasteride was linked with elevations in the phylum Bacteroidetes and in the family Prevotellaceae, and withdrawal was found to be correlated with decreases in the family Ruminococcaceae and the genera Oscillospira and Lachnospira [ 188 ]. In another study, socially stressed mice developing depression-like symptoms showed increases at the genus level of fecal Oscillospira . Interestingly, IV administration of anti-mouse IL-6 receptor antibody (MR16-1) normalized depression-like behavior and resulted in significant decrease in Oscillospira levels towards pre-stressor levels [ 3 ]. Moreover, increased number of Sutterella was reported in fecal samples [ 189 ] and intestinal biopsy samples of children with Autism spectrum disorder [ 190 ]. Additionally, elevated number of Staphylococcus and Sutterella were found in mice following social defeat stress. It is likely that Staphylococcus and Sutterella play a role in the depressive-like behavior via infection-induced inflammation. Interestingly, administration of anti-mouse IL-6 receptor antibody resulted in attenuation of elevated number of Staphylococcus and Sutterella following social defeat stress in mice [ 3 ].
These findings advocate that peripheral IL-6 may have a significant role in pathogenesis of MDD and blockade of IL-6 receptor in the periphery may exhibit rapid-onset effects by attenuating the altered composition of gut microbiota. Taking into account the role of gut-microbiota in immunomodulation, it is highly probable that gut-microbiota-brain- axis plays a role in anti-depressant actions of treatment with anti-IL-6 receptor [ 3 ]. A brief overview of the role of IL-6 together with gut microbiota in pathogenesis of depression is shown in Fig. 2 .
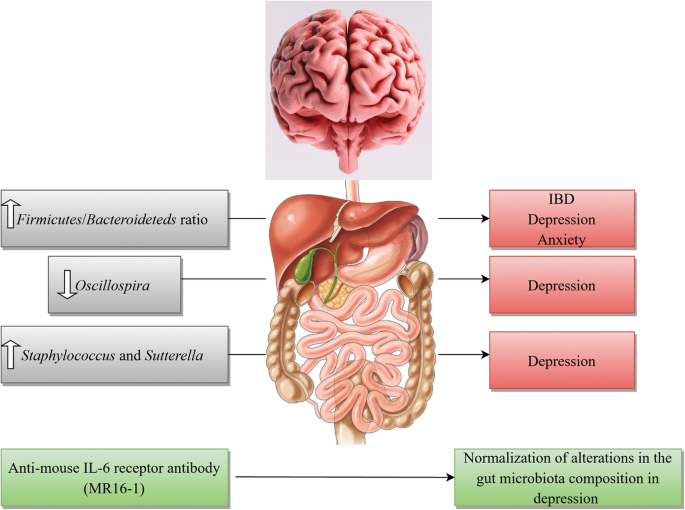
A brief overview of the role of IL-6 together with gut microbiota in pathogenesis of depression
Elevated levels of IL-6 in patients with COVID-19 infection
The world-wide effect of the coronavirus disease 2019 (COVID-19) pandemic is enormous and is not solely limited to the increased mortality and morbidity rates, but also extends into the mental health of the global population [ 191 ]. Considerable amount of clinical data is emerging regarding the manifestation of depression in patients during [ 192 , 193 , 194 ] and post-COVID 19 infection [ 192 , 193 , 194 , 195 ]. It is estimated that about 48% of confirmed COVID-19 cases displayed overt psychological symptoms such as depression and often expressed feelings of regret, loneliness, helplessness, and irritation [ 196 ].
There is growing body of literature regarding dual role of IL-6 in COVID-19 infection and depression [ 192 ]. Normal plasma levels of IL-6 in adults range between 1 and 10 pg/ml; whereas in a systemic inflammation this amount increases to several ng/ml [ 197 ] and even higher concentrations were reported in COVID-19 patients [ 198 ]. Indeed, cytokine release syndrome (CRS) is common in COVID-19 patients and increased levels of serum IL-6 have been identified to be significantly associated with acute respiratory distress syndrome (ARDS), respiratory failure, and poor disease outcome in numerous studies [ 192 , 199 , 200 , 201 ]. Studies suggest that new onset depression is most probably caused by inflammation initiated during the active phase of the infection leading to a cytokine surge [ 202 ]. Indeed, severe acute respiratory syndrome coronavirus 2 (SARS-CoV-2) infects primarily human monocytes and dendritic cells causing dendritic cell dysfunction, leading to T cell apoptosis and exhaustion; thus, contributing to the immunopathology [ 203 ]. Alpert et al. (2020) described two cases of COVID-19 patients with elevated amounts of IL-6 (25 pg/mL and 26.7 pg/mL respectively) who were diagnosed with major depressive disorder (according to the Diagnostic and Statistical Manual of Mental Disorder, 5th Edition (DSM-5)) during COVID 19 infection. Both patients’ depressive symptoms subsided about 6 weeks after initiation of anti-depressant pharmacotherapy and normalization of the inflammatory cytokines [ 192 ]. The authors concluded that lower cytokine activity ameliorates depressive symptoms as normalization of IL-6 plasma levels decreased depression with or without anti-depressants [ 192 ]. Moreover, Benedett et al. (2020) studied effects of treatment with cytokine-blocking agents on the psychopathological status of the patients with COVID-19 infection. Their results were in favor of the protective effects of treatment with cytokine-blocking agents in early phases of COVID-19 against the later onset of depression [ 204 ].
It is indeed crucial to maintain a multidisciplinary approach in management of the psychological effects of this debilitating pandemic. Treatment strategies addressing the immunopathology of SARS-CoV-2 infection will be promising during the acute phase of the disease [ 192 , 205 ]. Currently, there are few studies considering psychological and neuropsychiatric implications of COVID-19; however, it is very likely to expect an increased incidence of mental pathologies both during and post-COVID-19 infection.
Preclinical and clinical studies present strong evidence that inflammation is altered in a subset of patients with MDD and there is mounting body of literature for the role of pro-inflammatory cytokines namely IL-6 in pathophysiology of depression. Nevertheless, there still exists gap in our understanding of the mechanisms by which IL-6 signaling and its molecular components could possibly contribute to depression manifestation. A number of humanized monoclonal antibodies are undergoing clinical trials for potential pharmacotherapy of mood disorders. Biologics including IL-6 receptor antibodies or IL-6 antibodies are currently approved to treat inflammatory disorders such as RA and are undergoing clinical trials as a novel target for MDD treatment. However, these novel therapeutic targets may also raise the possibility of potential side effects. By investigating the interface of peripheral cytokines, namely IL-6 and brain cellular processes contributing to depression, one might be able to develop novel therapeutic options for treatment of mood disorders by sequestering and preventing this peripherally derived inflammatory marker from acting upon mood circuits in the CNS. In sum, therapeutic deficiency in treatment outcomes reflects the growing demand for revitalizing psychiatric therapeutics with novel options that could potentially open new avenues in treatment of this debilitating disorder and enhancement of patients’ quality of life.
Availability of data and materials
Not applicable.
Abbreviations
Anterior cingulate cortex
Acute respiratory distress syndrome
Blood-brain barrier
Central nervous system
Coronavirus disease 2019
Cytokine release syndrome
Conserved transcriptional response to adversity
Disease-modifying anti-rheumatic drugs
Diagnostic and Statistical Manual of Mental Disorder, 5th Edition
Electroconvulsive therapy
Glycoprotein 130
G-protein-coupled receptors
Hypothalamic-pituitary-adrenal
Indoleamine 2,3 dioxygenase
Interleukin
IL-6 receptor
Janus kinase/signal transducer and activator of transcription
- Major depressive disorder
Mitogen-activated protein kinase
Messenger RNA
Nuclear factor kappa-light-chain-enhancer of activated B cells
Prefrontal cortex
Rheumatoid arthritis
severe acute respiratory syndrome coronavirus 2
Serotonin transporter
Signal transducer and activator of transcription 3
Soluble IL-6R
Transforming growth factor
T helper type 2
T helper type 1
T helper cell
Tumor necrosis factor
Translocator protein
Regulatory T cells
Depression and Other Common Mental Disorders: Global Health Estimates. 2017. Geneva: World Health Organization, Licence: CC BY-NC-SA 3.0 IGO.
Liu Q, He H, Yang J, Feng X, Zhao F, Lyu J. Changes in the global burden of depression from 1990 to 2017: Findings from the Global Burden of Disease study. J Psychiatr Res. 2020;126:134–40.
Article PubMed Google Scholar
Zhang JC, Yao W, Dong C, Yang C, Ren Q, Ma M, Hashimoto K. Blockade of interleukin-6 receptor in the periphery promotes rapid and sustained antidepressant actions: a possible role of gut-microbiota-brain axis. Transl Psychiatry. 2017;7:e1138.
Article CAS PubMed PubMed Central Google Scholar
Sim K, Lau WK, Sim J, Sum MY, Baldessarini RJ. Prevention of Relapse and Recurrence in Adults with Major Depressive Disorder: Systematic Review and Meta-Analyses of Controlled Trials. Int J Neuropsychopharmacol. 2016;19:pyv076.
Hodes GE, Ménard C, Russo SJ. Integrating Interleukin-6 into depression diagnosis and treatment. Neurobiol Stress. 2016;4:15–22.
Article PubMed PubMed Central Google Scholar
Fonseka TM, McIntyre RS, Soczynska JK, Kennedy SH. Novel investigational drugs targeting IL-6 signaling for the treatment of depression. Expert Opin Investig Drugs. 2015;24:459–75.
Article CAS PubMed Google Scholar
Miller AH, Raison CL. The role of inflammation in depression: from evolutionary imperative to modern treatment target. Nat Rev Immunol. 2016;16:22–34.
Majd M, Hashemian F, Hosseini SM, Vahdat Shariatpanahi M. Sharifi A. A randomized, double-blind, placebo-controlled trial of celecoxib augmentation of sertraline in treatment of drug-naive depressed women: a pilot study. Iran J Pharm Res. 2015;14:891–9.
CAS PubMed PubMed Central Google Scholar
Wohleb ES, Franklin T, Iwata M, Duman RS. Integrating neuroimmune systems in the neurobiology of depression. Nat Rev Neurosci. 2016;17:497–511.
Zhao G, Liu X. Neuroimmune advance in depressive disorder. Adv Exp Med Biol. 2019;1180:85–98.
Lee CH, Giuliani F. The role of inflammation in depression and fatigue. Front Immunol. 2019;10:1696.
Liu CH, Zhang GZ, Li B, Li M, Woelfer M, Walter M, Wang L. Role of inflammation in depression relapse. J Neuroinflammation. 2019;16:90.
Cassano T, Calcagnini S, Carbone A, Bukke VN, Orkisz S, Villani R, Romano A, Avolio C, Gaetani S. Pharmacological treatment of depression in Alzheimer’s disease: a challenging task. Front Pharmacol. 2019;10:1067.
Hirschfield RM. History and evolution of the monoamine hypothesis of depression. J Clin Psychiatry. 2000;61:4–6.
Google Scholar
Massart R, Mongeau R, Lanfumey L. Beyond the monoaminergic hypothesis: neuroplasticity and epigenetic changes in a transgenic mouse model of depression. PhilosTrans R Soc Lond Ser B Biol Sci. 2012;367:2485–94.
Article CAS Google Scholar
Boku S, Nakagawa S, Toda H, Hishimoto A. Neural basis of major depressive disorder: Beyond monoamine hypothesis. Psychiatry Clin Neurosci. 2018;72:3–12.
Kopschina Feltes P, Doorduin J, Klein HC, Juárez-Orozco LE, Dierckx RA. Moriguchi- Jeckel CM, de Vries EF. Anti-inflammatory treatment for major depressive disorder: implications for patients with an elevated immune profile and non-responders to standard antidepressant therapy. J Psychopharmacol. 2017;31:1149–65.
Maes M, Van der Planken M, Stevens WJ, Peeters D, DeClerck LS, Bridts CH, Schotte C, Cosyns P. Leukocytosis, monocytosis and neutrophilia: hallmarks of severe depression. J Psychiatr Res. 1992;26:125–134.
Maes M, Lambrechts J, Bosmans E, Jacobs J, Suy E, Vandervorst C, de Jonckheere C, Minner B, Raus J. Evidence for a systemic immune activation during depression: results of leukocyte enumeration by flow cytometry in conjunction with monoclonal antibody staining. Psychol Med. 1992;22:45–53.
Maes M, Scharpé S, Meltzer HY, Bosmans E, Suy E, Calabrese J, Cosyns P. Relationships between interleukin-6 activity, acute phase proteins, and function of the hypothalamic- pituitary-adrenal axis in severe depression. Psychiatry Res. 1993;49:11–27.
Slavich GM, Irwin MR. From stress to inflammation and major depressive disorder: a social signal transduction theory of depression. Psychol Bull. 2014;140:774–815.
Irwin MR, Miller AH. Depressive disorders and immunity: 20 years of progress and discovery. Brain Behav Immun. 2007;21:374–83.
Henter ID, de Sousa RT, Gold PW, Brunon AR, Zarate CA, Machado-Vieira R. Mood therapeutics: novel pharmacological approaches for treating depression. Expert Rev Clin Pharmacol. 2017;10:153-166.
Himmerich H, Patsalos O, Lichtblau N, Ibrahim MAA, Dalton B. Cytokine research in depression: principles, challenges, and open questions. Front Psychiatry. 2019;7(10):30.
Article Google Scholar
Zou W, Feng R, Yang Y. Changes in the serum levels of inflammatory cytokines in antidepressant drug-naïve patients with major depression. PLoS One. 2018;13:e0197267.
Article PubMed PubMed Central CAS Google Scholar
Miller AH, Maletic V, Raison CL. Inflammation and its discontents: the role of cytokines in the pathophysiology of major depression. Biol Psychiatr. 2009;65:732–41.
Horowitz MA, Zunszain PA. Neuroimmune and neuroendocrine abnormalities in depression: two sides of the same coin. Ann N Y Acad Sci. 2015;1351:68–79.
Raison CL. Miller AH. Is depression an inflammatory disorder? Curr Psychiatry Rep. 2011;13:467–75.
Young JJ, Bruno D. Pomara N. A review of the relationship between proinflammatory cytokines and major depressive disorder. J Affect Disord. 2014;169:15–20.
Kim YK, Na KS, Shin KH, Jung HY, Choi SH, Kim JB. Cytokine imbalance in the pathophysiology of major depressive disorder. Prog Neuropsychopharmacol Biol Psychiatry. 2007;31:1044–53.
Himmerich H, Kohls E, Hegerl U, Rummel-Kluge C. Prädiktive Faktoren der Depression und ihrer Therapie. Der Nervenarzt. 2014;85:1249–54.
Muñoz RF, Cuijpers P, Smit F, Barrera AZ, Leykin Y. Prevention of major depression. Ann Rev Clin Psychol. 2010;6:181–212.
Brebner K, Hayley S, Zacharko R, Merali Z, Anisman H. Synergistic effects of interleukin-1β, interleukin-6, and tumor necrosis factor-α: Central monoamine, corticosterone, and behavioral variations. Neuropsychopharmacology. 2000;22:566–80.
Farooq RK, Asghar K, Kanwal S, Zulqernain A. Role of inflammatory cytokines in depression: focus on interleukin-1β. Biomed Rep. 2017;6:15–20.
Felger JC, Lotrich FE. Inflammatory cytokines in depression: neurobiological mechanisms and therapeutic implications. Neuroscience. 2013;246:199–229.
Black C, Miller BJ. Meta-analysis of cytokines and chemokines in suicidality: distinguishing suicidal versus nonsuicidal patients. Biol Psychiatry. 2015;78:28–37.
Goldsmith DR, Rapaport MH. Miller BJ. A meta-analysis of blood cytokine network alterations in psychiatric patients: comparisons between schizophrenia, bipolar disorder and depression. Mol Psychiatry. 2016;21:1696–709.
Dowlati Y, Herrmann N, Swardfager W, Liu H, Sham L, Reim EK. Lanctôt KL. A meta-analysis of cytokines in major depression. Biol Psychiatry. 2010;67:446–57.
Strawbridge R, Arnone D, Danese A, Papadopoulos A, Herane Vives A, Cleare AJ. Inflammation and clinical response to treatment in depression: A meta-analysis. Eur Neuropsychopharmacol. 2015;25:1532–43.
Fan N, Luo Y, Ou Y, He H. Altered serum levels of TNF-α, IL-6, and IL-18 in depressive disorder patient. Hum Psychopharmacol. 2017;32(4) https://doi.org/10.1002/hup.2588 .
Nishuty NL, Khandoker MH, Karmoker JR, Ferdous S, Shahriar M, Qusar S, Islam S. Kadir MF, Islam R. Evaluation of serum interleukin-6 and C-reactive protein levels in drug-naïve major depressive disorder patient. cureus. 2019;11:e3868.
PubMed PubMed Central Google Scholar
Ramesh G, MacLean AG, Philipp MT. Cytokines and chemokines at the crossroads of neuroinflammation, neurodegeneration. and neuropathic pain. Mediators Inflamm. 2013;2013:480739.
PubMed Google Scholar
Arango Duque G, Descoteaux A. Macrophage cytokines: involvement in immunity and infectious diseases. Front Immunol. 2014;7(5):491.
Galic MA, Riazi K, Pittman QJ. Cytokines and brain excitability. Front Neuroendocrinol. 2012;33:116–25.
Wilson CJ, Finch CE, Cohen HJ. Cytokines and cognition--the case for a head-to-toe inflammatory paradigm. J Am Geriatr Soc. 2002;50:2041–56.
Hopkins SJ. Central nervous system recognition of peripheral inflammation: a neural. hormonal collaboration. Acta Biomed. 2007;78:231–47.
Capuron L, Miller AH. Immune system to brain signaling: neuropsychopharmacological implications. Pharmacol Ther. 2011;130:226–38.
Caldwell AB, Cheng Z, Vargas JD, Birnbaum HA, Hoffmann A. Network dynamics determine the autocrine and paracrine signaling functions of TNF. Genes Dev. 2014;28:2120–33.
Miller AH, Haroon E, Raison CL, Felger JC. Cytokine targets in the brain: impact on neurotransmitters and neurocircuits. Depress Anxiety. 2013;30:297–306.
Perskidskii IV, Barshtein IA. Biological manifestations of the tumor necrosis factor effect and its role in the pathogenesis of various diseases. Arkh Patol. 1992;54:5–10.
Zhang JM, An J. Cytokines, inflammation. and pain. Int Anesthesiol Clin. 2007;45:27–37.
Kicielinska J, Pajtasz-Piasecka E. The role of IL-10 in the modulation of the immune response in normal conditions and the tumor environment. Postepy Higieny Med Doświadczal. 2014;68:879–92.
Munk ME, Emoto M. Functions of T-cell subsets and cytokines in mycobacterial infections. Eur Respir J Suppl. 1995;20:668s–−675s.
CAS PubMed Google Scholar
Krumm B, Xiang Y, Deng J. Structural biology of the IL-1 superfamily: key cytokines in the regulation of immune and inflammatory responses. Protein Sci. 2014;23:526–38.
Tilg H, Peschel C. Interferon-alpha and its effects on the cytokine cascade: a pro- and anti-inflammatory cytokine. Leukem Lymphoma. 1996;23:55–60.
Klimpel GR. Soluble factor(s) from LPS-activated macrophages induce cytotoxic T cell differentiation from alloantigen-primed spleen cells. J Immunol. 1980;125:1243–9.
Spooren A, Kolmus K, Laureys G, Clinckers R, De Keyser J, Haegeman G, Gerlo S. Interleukin-6. a mental cytokine. Brain Res Rev. 2011;67:157–83.
Yamasaki K, Taga T, Hirata Y, Yawata H, Kawanishi Y, Seed B, Taniguchi T, Hirano T, Kishimoto T. Cloning and expression of the human interleukin-6 (BSF-2/IFN beta 2) receptor. Science. 1988;241:825–8.
Hibi M, Murakami M, Saito M, Hirano T, Taga T, Kishimoto T. Molecular cloning and expression of an IL-6 signal transducer, gp130. Cell. 1990;63:1149–57.
Fuster JJ, Walsh K. The good, the bad, and the ugly of interleukin-6 signaling. EMBO J. 2014;33:1425–7.
Schett G. Physiological effects of modulating the interleukin-6 axis. Rheumatology. 2018;57:ii43–ii50.
Mullberg J, Schooltink H, Stoyan T, Gunther M, Graeve L, Buse G, Mackiewicz A. Heinrich PC, Rose-John S. The soluble interleukin-6 receptor is generated by shedding. Eur J Immunol. 1993;23:473–80.
Wolf J, Rose-John S, Garbers C. Interleukin-6 and its receptors: a highly regulated and dynamic system. Cytokine. 2014;70:11–20.
Scheller J, Chalaris A, Schmidt-Arras D, Rose-John S. The pro- and anti-inflammatory properties of the cytokine interleukin-6. Biochim Biophys Acta. 2011;1813:878–88.
Hunter CA, Jones SA. IL-6 as a keystone cytokine in health and disease. Nat Immunol. 2015;16:448–57.
Hegde S, Pahne J, Smola-Hess S. Novel immunosuppressive properties of interleukin- 6 in dendritic cells: inhibition of NF-kB binding activity and CCR7 expression. FASEB J. 2004;18:1439–41.
Kimura A, Kishimoto T. IL-6: regulator of Treg/Th17 balance. Eur J Immunol. 2010;40:1830–5.
Mangan PR, Harrington LE, O’Quinn DB, Helms WS, Bullard DC, Elson CO, Hatton RD, Wahl SM, Schoeb TR, Weaver CT. Transforming growth factor-beta induces development of the T(H)17 lineage. Nature. 2006;441:231–4.
Bettelli E, Carrier Y, Gao W, Korn T, Strom TB, Oukka M, Weiner HL, Kuchroo VK. Reciprocal developmental pathways for the generation of pathogenic effector TH17 and regulatory T cells. Nature. 2006;441:235–8.
Iwakura Y, Ishigame H, Saijo S, Nakae S. Functional specialization of interleukin-17 family members. Immunity. 2011;34:149–62.
Dominitzki S, Fantini MC, Neufert C, Nikolaev A, Galle PR, Scheller J, Monteleone G, Rose-John S, Neurath MF, Becker C. Cutting edge: trans-signaling via the soluble IL-6R abrogates the induction of FoxP3 in naïve CD4+CD25 T cells. J Immunol. 2007;179:2041–5.
Milovan BM, Ivan J, Gordana R, Jelena P, Slavica MJ, Nebojsa A, Lukic ML. Interleukin-6 in schizophrenia—is there a therapeutic relevance? Front Psychiatry. 2017;8:221.
Milenkovic VM, Stanton EH, Nothdurfter C, Rupprecht R, Wetzel CH. The role of chemokines in the pathophysiology of major depressive disorder. Int J Mol Sci. 2019;20:2283.
Article CAS PubMed Central Google Scholar
Zlotnik A, Yoshie O. The chemokine superfamily revisited. Immunity. 2012;36:705–16.
Le Thuc O, Blondeau N, Nahon JL, Rovère C. The complex contribution of chemokines to neuroinflammation: switching from beneficial to detrimental effects. Ann N Y Acad Sci. 2015;1351:127–40.
Article PubMed CAS Google Scholar
Deshmane SL, Kremlev S, Amini S, Sawaya BE. Monocyte chemoattractant protein-1 (MCP-1): an overview. J Interferon Cytokine Res. 2009;29:313–26.
Stuart MJ, Baune BT. Chemokines and chemokine receptors in mood disorders, schizophrenia, and cognitive impairment: a systematic review of biomarker studies. Neurosci Biobehav Rev. 2014;42:93–115.
Ślusarczyk J, Trojan E, Głombik K, Budziszewska B, Kubera M, Lasoń W, Popiołek- Barczyk K, Mika J, Wędzony K, Basta-Kaim A. Prenatal stress is a vulnerability factor for altered morphology and biological activity of microglia cells. Front Cell Neurosci. 2015;9:82.
Trojan E, Ślusarczyk J, Chamera K, Kotarska K, Głombik K, Kubera M, Basta-Kaim A. The modulatory properties of chronic antidepressant drugs treatment on the brain chemokine – chemokine receptor network: a molecular study in an animal model of depression. Front Pharmacol. 2017;8:779.
Simon NM, McNamara K, Chow CW, Maser RS, Papakostas GI, Pollack MH, Nierenberg AA, Fava M. Wong KK. A detailed examination of cytokine abnormalities in major depressive disorder. Eur Neuropsychopharmacol. 2008;18:230–3.
Piletz JE, Halaris A, Iqbal O, Hoppensteadt D, Fareed J, Zhu H, Sinacore J, Devane CL. Pro- inflammatory biomakers in depression: treatment with venlafaxine. World J Biol Psychiatry. 2009;10:313–23.
De la Peña FR, Cruz-Fuentes C, Palacios L, Girón-Pérez MI, Medina-Rivero E. Ponce- Regalado MD, Alvarez-Herrera S, Pérez-Sánchez G, Becerril-Villanueva E, Maldonado-García JL, Jiménez-Martínez MC, Pavón L. Serum levels of chemokines in adolescents with major depression treated with fluoxetine. World J Psychiatry. 2020;10:175–86.
Romero-Sanchiz P, Nogueira-Arjona R, Araos P, Serrano A, Barrios V, Argente J, Garcia- Marchena N, Lopez-Tellez A, Rodriguez-Moreno S, Mayoral F, et al. Variation in chemokines plasma concentrations in primary care depressed patients associated with Internet-based cognitive-behavioral therapy. Sci Rep. 2020;10:1078.
Leighton SP, Nerurkar L, Krishnadas R, Johnman C, Graham GJ, Cavanagh J. Chemokines in depression in health and in inflammatory illness: a systematic review and meta- analysis. Mol Psychiatry. 2018;23:48–58.
Ślusarczyk J, Trojan E, Chwastek J, Głombik K, Basta-Kaim AA. Potential Contribution of Chemokine Network Dysfunction to the Depressive Disorders. Curr Neuropharmacol. 2016;14:705–20.
Sasayama D, Hattori K, Wakabayashi C, Teraishi T, Hori H, Ota M, Yoshida S, Arima K, Higuchi T, Amano N, et al. Increased cerebrospinal fluid interleukin-6 levels in patients with schizophrenia and those with major depressive disorder. J Psychiatr Res. 2013;47:401–6.
Boufidou F, Lambrinoudaki I, Argeitis J, Zervas IM, Pliatsika P, Leonardou AA. Petropoulos G, Hasiakos D, Papadias K, Nikolaou C. CSF and plasma cytokines at delivery and postpartum mood disturbances. J Affect Disord. 2009;115:287–92.
Schmidt FM, Weschenfelder J, Sander C, Minkwitz J, Thormann J, Chittka T, Mergl R, Kirkby KC, Faßhauer M, Stumvoll M, et al. Inflammatory cytokines in general and central obesity and modulating effects of physical activity. PLoS ONE. 2015;10:e0121971.
Bastard JP, Jardel C, Delattre J, Hainque B, Bruckert E, Oberlin F. Evidence for a link between adipose tissue interleukin-6 content and serum C-reactive protein concentrations in obese subjects. Circulation. 1999;99:2221–2.
Wellen KE, Hotamisligil GS. Obesity-induced inflammatory changes in adipose tissue. J Clin Invest. 2003112:1785–8.
Sweat V, Starr V, Bruehl H, Arentoft A, Tirsi A, Javier E. Convit A. C-reactive protein is linked to lower cognitive performance in overweight and obese women. Inflammation. 2008;31:198–207.
Capuron L, Su S, Miller AH, Bremner JD, Goldberg J, Vogt GJ, Maisano C, Jones L. Murrah NV, Vaccarino V. Depressive symptoms and metabolic syndrome: is inflammation the underlying link? Biol Psychiatry. 2008;64:896–900.
Hou R, Garner M, Holmes C, Osmond C, Teeling J, Lau L, Baldwin DS. Peripheral inflammatory cytokines and immune balance in Generalised Anxiety Disorder: Case- controlled study. Brain Behav Immun. 2017;62:212–8.
Costello H, Gould RL, Abrol E, Howard R. Systematic review and meta-analysis of the association between peripheral inflammatory cytokines and generalised anxiety disorder. BMJ Open. 2019;9:e027925.
Vieira MMM, Ferreira TB, Pacheco PAF, Barros PO, Almeida CRM, Araújo-Lima CF, Silva-Filho RG, Hygino J, Andrade RM, Linhares UC, et al. Enhanced Th17 phenotype in individuals with generalized anxiety disorder. J Neuroimmunol. 2010;229:212–8.
Gray SM, Bloch MH. Systematic review of proinflammatory cytokines in obsessive- compulsive disorder. Curr Psychiatr Rep. 2012;14:220–8.
Hori H, Kim Y. Inflammation and post-traumatic stress disorder. PCN Psychiatry and Clinical Neurosciences. 2019;73:143–53.
Renna ME, O’Toole MS, Spaeth PE, Lekander M, Mennin DS. The association between anxiety, traumatic stress, and obsessive-compulsive disorders and chronic inflammation: a systematic review and meta-analysis. Depress Anxiety. 2018;35:1081–94.
Irwin MR. Why sleep is important for health: a psychoneuroimmunology perspective. Annu Rev Psychol. 2015;66:143–72.
Köhler CA, Freitas TH, Stubbs B, Maes M, Solmi M, Veronese N, de Andrade NQ, Morris G, Fernandes BS, Brunoni AR, et al. Peripheral alterations in cytokine and chemokine levels after antidepressant drug treatment for major depressive disorder: systematic review and meta-analysis. Mol Neurobiol. 2018;55:4195–206.
Himmerich H, Minkwitz J, Kirkby K. Weight gain and metabolic changes during treatment with antipsychotics and antidepressants. Endocr Metabol Immune Disorder Drug Targets. 2015;15:252–60.
Himmerich H, Koethe D, Schuld A, Yassouridis A, Pollmacher T. Plasma levels of leptin and endogenous immune modulators during treatment with carbamazepine or lithium. Psychopharmacology. 2005;179:447–51.
Haapakoski R, Mathieu J, Ebmeier KP, Alenius H, Kivimaki M. Cumulative meta- analysis of interleukins 6 and 1beta, tumour necrosis factor alpha and C-reactive protein in patients with major depressive disorder. Brain Behav Immun. 2015;49:206–15.
Khandaker GM, Pearson RM, Zammit S, Lewis G, Jones PB. Association of serum interleukin 6 and C-reactive protein in childhood with depression and psychosis in young adult life: a population-based longitudinal study. JAMA Psychiatry. 2014;71:1121–8.
Lanquillon S, Krieg JC, Bening-Abu-Shach U, Vedder H. Cytokine production and treatment response in major depressive disorder. Neuropsychopharmacology. 2000;22:370–9.
Mutlu O, Gumuslu E, Ulak G, Celikyurt IK, Kokturk S, Kir HM, Akar F, Erden F. Effects of fluoxetine, tianeptine and olanzapine on unpredictable chronic mild stress-induced depression-like behavior in mice. Life Sci. 2012;91:1252–62.
Pan Y, Zhang WY, Xia X, Kong LD. Effects of icariin on hypothalamic-pituitary- adrenal axis action and cytokine levels in stressed Sprague-Dawley rats. Biol Pharm Bull. 2006;29:2399–403.
Himmerich H, Fischer J, Bauer K, Kirkby KC, Sack U, Krugel U. Stress-induced cytokine changes in rats. Eur Cytokine Network. 2013;24:97–103.
Farooq RK, Isingrini E, Tanti A, Le Guisquet AM, Arlicot N, Minier F, Leman S, Chalon S, Belzung C. Camus V. Is unpredictable chronic mild stress (UCMS) a reliable model to study depression-induced neuroinflammation? Behav Brain Res. 2012;231:130–7.
Monje FJ, Cabatic M, Divisch I, Kim EJ, Herkner KR, Binder BR, Pollak DD. Constant darkness induces IL-6-dependent depression-like behavior through the NF-kappaB signaling pathway. J Neurosci. 2011;31:9075–83.
Russo SJ, Murrough JW, Han MH, Charney DS, Nestler EJ. Neurobiology of resilience. Nat Neurosci. 2012;15:1475–84.
Hodes GE, Pfau ML, Leboeuf M, Golden SA, Christoffel DJ, Bregman D, Rebusi N, Heshmati M, Aleyasin H, Warren BL, et al. Individual differences in the peripheral immune system promote resilience versus susceptibility to social stress. Proc Natl Acad Sci U S A. 2014;111:16136–41.
Krishnan V. Molecular adaptations underlying susceptibility and resistance to social defeat in brain reward regions. Cell. 2007;131:391–404.
Yang C, Shirayama Y, Zhang JC, Ren Q, Hashimoto K. Peripheral interleukin-6 promotes resilience versus susceptibility to inescapable electric stress. Acta Neuropsychiatr. 2015;27:312–6.
Wang M, Wei J, Yang X, Ni P, Wang Y, Zhao L, Deng W, Guo W, Wang Q, Li T, et al. The level of IL-6 was associated with sleep disturbances in patients with major depressive disorder. Neuropsychiatric Disease and Treatment. 2019;15:1695–700.
Howren MB, Lamkin DM, Suls J. Associations of depression with C-reactive protein, IL-1, and IL-6: a meta-analysis. Psychosom Med. 2009;71:171–86.
Liu Y, Ho RC, Mak A. Interleukin (il)-6, tumour necrosis factor alpha (tnf-alpha) and soluble interleukin-2 receptors (sil-2r) are elevated in patients with major depressive disorder: A meta-analysis and meta-regression. J Affect Disord. 2012;139:230–9.
Chocano-Bedoya PO, Mirzaei F, O’Reilly EJ, Lucas M, Okereke OI, Hu FB, Rimm EB, Ascherio A. C-reactive protein, interleukin-6, soluble tumor necrosis factor alpha receptor 2 and incident clinical depression. J Affect Disord. 2014;163:25–32.
Rudolf S, Greggersen W, Kahl KG, Huppe M, Schweiger U. Elevated il-6 levels in patients with atypical depression but not in patients with typical depression. Psychiatry Res. 2014;217:34–8.
Rush G, O’Donovan A, Nagle L, Conway C, McCrohan A, O’Farrelly C, Lucey JV, Malone KM. Alteration of immune markers in a group of melancholic depressed patients and their response to electroconvulsive therapy. J Affect Disord. 2016;205:60–8.
Yang C, Tiemessen KM, Bosker FJ, Wardenaar KJ, Lie J, Schoevers RA. Interleukin, tumor necrosis factor-alpha and c-reactive protein profiles in melancholic and non-melancholic depression: A systematic review. J Psychosom Res. 2018;111:58–68.
Maes M, Bosmans E, De Jongh R, Kenis G, Vandoolaeghe E, Neels H. Increased serum IL- 6 and IL-1 receptor antagonist concentrations in major depression and treatment resistant depression. Cytokine. 1997;9:853–8.
Lichtblau N, Schmidt FM, Schumann R, Kirkby KC, Himmerich H. Cytokines as biomarkers in depressive disorder: current standing and prospects. Int Rev Psychiatry. 2013;25:592–603.
Cowen PJ, Browning M. What has serotonin to do with depression? World Psychiatry. 2015;14:158–60.
Albert PR, Benkelfat C, Descarries L. The neurobiology of depression—revisiting the serotonin hypothesis. I. Cellular and molecular mechanisms. Philos Trans R Soc Lond B Biol Sci. 2012;367:2378–81.
Zhu CB, Carneiro AM, Dostmann WR, Hewlett WA. Blakely RD. p38 MAPK Activation Elevates Serotonin Transport Activity via a Trafficking-independent, Protein Phosphatase 2A-dependent Proces. The Journal of Biological Chemistry. 2005;280:15649–58.
Zhu CB, Blakely RD, Hewlett WA. The proinflammatory cytokines interleukin-1beta and tumor necrosis factor-alpha activate serotonin transporters. Neuropsychopharmacology. 2006;31:2121–31.
Kong E, Sucic S, Monje FJ, Reisinger SN, Savalli G, Diao W, Khan D, Ronovsky M, Cabatic M, Koban F, et al. STAT3 controls IL6-dependent regulation of serotonin transporter function and depression-like behavior. Sci Rep. 2015;5:9009.
Baudry A, Pietri M, Launay JM, Kellermann O, Schneider B. Multifaceted regulations of the serotonin transporter: impact on antidepressant response. Front Neurosci. 2019;13:91.
Zalcman S, Green-Johnson JM, Murray L, Nance DM, Dyck D, Anisman H, Greenberg AH. Cytokine-specific central monoamine alterations induced by interleukin-1, -2 and – 6. Brain Res. 1994;643:40–9.
Day JS, O’Neill E, Cawley C, Aretz NK, Kilroy D, Gibney SM, Harkin A, Conor TJ. Noradrenaline acting on astrocyticβ2-adrenoceptors induces neurite outgrowth in primary cortical neurons. Neuropharmacology. 2014;77:234–48.
Müller N, Myint AM, Krause D, Weidinger E, Schwarz MJ. Anti-inflammatory treatment in schizophrenia. Prog Neuropsychopharmacol Biol Psychiatry. 2013;42:146–53.
Monje ML, Toda H, Palmer TD. Inflammatory blockade restores adult hippocampal neurogenesis. Science. 2003;302:1760–5.
Khairova RA, Machado-Vieira R, Du J. Manji HK. A potential role for pro-inflammatory cytokines in regulating synaptic plasticity in major depressive disorder. Int J Neuropsychopharmacol. 2009;12:561–78.
Alexander GE, Crutcher MD, DeLong MR. Basal ganglia-thalamocortical circuits: parallel substrates for motor, oculomotor, “prefrontal” and “limbic” functions. Progress Brain Res. 1991;85:119–46.
Eisenberger NI, Lieberman MD. Why rejection hurts: a common neural alarm system for physical and social pain. Trends Cogn Sci. 2004;8:294–300.
Rothaug M, Becker-Pauly C, Rose-John S. The role of interleukin-6 signaling in nervous tissue. Biochim Biophys Acta. 2016;1863:1218–27.
Campbell IL, Abraham CR, Masliah E, Kemper P, Inglis JD, Oldstone MB, Mucke L. Neurologic disease induced in transgenic mice by cerebral overexpression of interleukin 6. Proc Natl Acad Sci U S A. 1993;90:10061–5.
Setiawan E, Attwells S, Wilson AA, Mizrahi R, Rusjan PM, Miler L, Xu C, Sharma S, Kish S, Houle S, et al. Association of translocator protein total distribution volume with duration of untreated major depressive disorder: a cross-sectional study. The Lancet Psychiatry. 2018;5:339–47.
Setiawan E, Wilson AA, Mizrahi R, Rusjan PM, Miler L, Rajkowska G, Suridjan I, Kennedy JL, Rekkas PV, Houle S, et al. Role of translocator protein density, a marker of neuroinflammation, in the brain during major depressive episodes. JAMA Psychiatry. 2015;72:268–75.
Holmes SE, Hinz R, Conen S, Gregory CJ, Matthews JC, Anton-Rodriguez JM, Gerhard A, Talbot PS. Elevated translocator protein in anterior cingulate in major depression and a role for inflammation in suicidal thinking: a positron emission tomography study. Biol Psychiatry. 2018;83:61–9.
Richards EM, Zanotti-Fregonara P, Fujita M, Newman L, Farmer C, Ballard ED, Machado- Vieira R, Yuan P, Niciu MJ, Lyoo CH, et al. PET radioligand binding to translocator protein (TSPO) is increased in unmedicated depressed subjects. EJNMMI Res. 2018;8:57.
Li H, Sagar AP, Kéri S. Translocator protein (18kDa TSPO) binding, a marker of microglia, is reduced in major depression during cognitive-behavioral therapy. Prog Neuropsychopharmacol Biol Psychiatry. 2018;83:1–7.
Kakeda S, Watanabe K, Katsuki A, Sugimoto K, Igata N, Ueda I, Igata R, Abe O, Yoshimura R, Korogi Y. Relationship between interleukin (IL)-6 and brain morphology in drug-naïve, first-episode major depressive disorder using surface-based morphometry. Sci Rep. 2018;8:10054.
Frodl T, Carballedo A, Hughes MM, Saleh K, Fagan A, Skokauskas N, McLoughlin DM, Meaney J, O'Keane V, Connor TJ. Reduced expression of glucocorticoid-inducible genes GILZ and SGK-1: high IL-6 levels are associated with reduced hippocampal volumes in major depressive disorder. Transl Psychiatry. 2012;2:e88.
Campbell S, Macqueen G. The role of the hippocampus in the pathophysiology of major depression. J Psychiatry Neurosci. 2004;29:417–26.
MacQueen GM, Campbell S, McEwen BS, Macdonald K, Amano S, Joffe RT, Nahmias C, Young LT. Course of illness, hippocampal function, and hippocampal volume in major depression. Proc Natl Acad Sci U S A. 2003;100:1387-1392.
Frodl T, Meisenzahl EM, Zetzsche T. Born C, Groll C, Jäger M, Leinsinger G, Bottlender R, Hahn K, Möller HJ. Hippocampal changes in patients with a first episode of major Depression. Am J Psychiatry. 2002;159:1112–8.
Rajkowska G. Postmortem studies in mood disorders indicate altered numbers of neurons and glial cells. Biol Psychiatry. 2000;48:766–7.
Cameron HA, McKay RD. Restoring production of hippocampal neurons in old age. Nat Neurosci. 1999;2:894–7.
Young EA, Haskett RF, Grunhaus L, Pande A, Weinberg VM, Watson SJ, Akil H. Increased evening activation of the hypothalamic-pituitary-adrenal axis in depressed patients. Arch Gen Psychiatry. 1994;51:701–7.
Sapolsky RM. The possibility of neurotoxicity in the hippocampus in major depression: a primer on neuron death. Biol Psychiatry. 2000 Oct 15;48(8):755–65.
McEwen BS. The neurobiology of stress: from serendipity to clinical relevance. Brain Res. 2000;886:172–89.
Yang C, Hashimoto K. Peripheral IL-6 signaling: a promising therapeutic target for depression? Expert Opin Investig Drugs. 2015;24:989–90.
Chourbaji S, Urani A, Inta I, Sanchis-Segura C, Brandwein C, Zink M, Schwaninger M, Gass P. IL-6 knockout mice exhibit resistance to stress-induced development of depression-like behaviors. Neurobiol Dis. 2006;23:587–94.
Ting EYC, Yang AC, Tsai AJ. Role of interleukin-6 in depressive disorder. Int J Mol Sci. 2020;21:2194.
Loppnow H, Zhang L, Buerke M, Lautenschläger M, Chen L, Frister A, Schlitt A, Luther T, Song N, Hofmann B, et al. Statins potently reduce the cytokine-mediated IL-6 release in SMC/MNC cocultures. J Cell Mol Med. 2011;15:994–1004.
Kang HJ, Bae KY, Kim SW, Kim JT, Park MS, Cho KH, Kim JM. Effects of interleukin-6, interleukin-18, and statin use, evaluated at acute stroke, on post-stroke depression during 1- year follow-up. Psychoneuroendocrinology. 2016;72:156–60.
Burmester GR, Rigby WF, van Vollenhoven RF, Kay J, Rubbert-Roth A, Kelman A, Dimonaco S, Mitchell N. Tocilizumab in early progressive rheumatoid arthritis: FUNCTION, a randomised controlled trial. Ann Rheum Di. 2016;75:1081-1091.
Yazici Y, Curtis JR, Ince A, Baraf H, Malamet RL, Teng LL, Kavanaugh A. Efficacy of tocilizumab in patients with moderate to severe active rheumatoid arthritis and a previous inadequate response to disease-modifying antirheumatic drugs: the ROSE study. Ann Rheum Dis. 2012;71:198–205.
Kaneko A. Tocilizumab in rheumatoid arthritis: efficacy, safety and its place in therapy. Ther Adv Chronic Dis. 2013;4:15–21.
Scott LJ. Tocilizumab: a review in rheumatoid arthritis. Drugs. 2017;77:1865–79.
Haraoui B, Casado G, Czirják L, Taylor A, Dong L, Button P, Luder Y, Caporali R. Tocilizumab patterns of use, effectiveness, and safety in patients with rheumatoid arthritis: final results from a set of multi-national non-interventional studies. Rheumatol Ther. 2019;6:231–43.
Turnier JL, Brunner HI. Tocilizumab for treating juvenile idiopathic arthritis. Expert Opin Biol Ther. 2016;16:559–66.
Machado SH, Xavier RM. Safety of tocilizumab in the treatment of juvenile idiopathic arthritis. Expert Opin Drug Saf. 2017;16:493–500.
Shepherd J, Cooper K, Harris P, Picot J, Rose M. The clinical effectiveness and cost- effectiveness of abatacept, adalimumab, etanercept and tocilizumab for treating juvenile idiopathic arthritis: a systematic review and economic evaluation. Health Technol Assess. 2016;20:1–222.
Brod SA, Bauer VL. Ingested (oral) tocilizumab inhibits EAE. Cytokine. 2014;68:86–93.
Nishimoto N, Kanakura Y, Aozasa K, Johkoh T, Nakamura M, Nakano S, Nakano N, Ikeda Y, Sasaki T, Nishioka K, et al. Humanized anti-interleukin-6 receptor antibody treatment of multicentric Castleman disease. Blood. 2005;106:2627–32.
Kappelmann N, Lewis G, Dantzer R, Jones PB, Khandaker GM. Antidepressant activity of anti-cytokine treatment: a systematic review and meta-analysis of clinical trials of chronic inflammatory conditions. Mol Psychiatry. 2018;23:335–43.
Taylor PC, Schiff MH, Wang Q, Jiang Y, Zhuang Y, Kurrasch R, Daga S, Rao R, Tak PP, Hsu B. Efficacy and safety of monotherapy with sirukumab compared with adalimumab monotherapy in biologic-naïve patients with active rheumatoid arthritis (SIRROUND-H): a randomised, double-blind, parallel-group, multinational, 52-week, phase 3 study. Annals of the Rheumatic Diseases. 2018;77:658–66.
Zhou AJ, Lee Y, Salvadore G, Hsu B, Fonseka TM, Kennedy SH, McIntyre RS. Sirukumab: a potential treatment for mood disorders? Adv Ther. 2017;34:78–90.
Sun Y, Wang D, Salvadore G, Hsu B, Curran M, Casper C, Vermeulen J, Kent JM, Singh J, Drevets WC, et al. The effects of interleukin-6 neutralizing antibodies on symptoms of depressed mood and anhedonia in patients with rheumatoid arthritis and multicentric castleman’s disease. Brain Behav. Immun. 2017;66:156–64.
Wittenberg GM, Stylianou A, Zhang Y, Sun Y, Gupta A, Jagannatha PS, Wang D, Hsu B, Curran ME, Khan S, et al. Effects of immunomodulatory drugs on depressive symptoms: A mega-analysis of randomized, placebo-controlled clinical trials in inflammatory disorders. Mol Psychiatry. 2020;25:1275–85.
Guinane CM, Cotter PD. Role of the gut microbiota in health and chronic gastrointestinal disease: understanding a hidden metabolic organ. Therap Adv Gastroenterol. 2013;6:295–308.
Sharon G, Sampson TR, Geschwind DH, Mazmanian SK. The central nervous system and the gut microbiome. Cell. 2016;167:915–32.
Cheung SG, Goldenthal AR, Uhlemann AC, Mann JJ, Miller JM, Sublette ME. Systematic review of gut microbiota and major depression. Front Psychiatry. 2019;10:34.
Kelly JR, Clarke G, Cryan JF, Dinan TG. Brain-gut-microbiota axis: challenges for translation in psychiatry. Ann Epidemiol. 2016;26:366–72.
Fung T, Olson C, Hsiao E. Interactions between the microbiota, immune and nervous systems in health and disease. Nat Neurosci. 2017;20:145–55.
Jiang H, Ling Z, Zhang Y, Mao H, Ma Z, Yin Y, Wang W, Tang W, Tan Z, Shi J, Li L, Ruan B. Altered fecal microbiota composition in patients with major depressive disorder. Brain Behav Immun. 2015;48:186–94.
Zheng P, Zeng B, Zhou C, Liu M, Fang Z, Xu X, Zeng L, Chen J, Fan S. Du X. Gut microbiome remodeling induces depressive-like behaviors through a pathway mediated by the host's metabolism. Mol Psychiatry. 2016;21:786–96.
Schirmer M, Smeekens SP, Vlamakis H, Jaeger M, Oosting M, Franzosa EA, Ter Horst R, Jansen T, Jacobs L, Bonder MJ, et al. Linking the human gut microbiome to inflammatory cytokine production capacity. Cell. 2016;167:1125–36 e8.
O'Mahony L, McCarthy J, Kelly P, Hurley G, Luo F, Chen K, O'Sullivan GC, Kiely B, Collins JK, Shanahan F, et al. Lactobacillus and bifidobacterium in irritable bowel syndrome: symptom responses and relationship to cytokine profiles. Gastroenterology. 2005;128:541–51.
Rinninella E, Raoul P, Cintoni M, Franceschi F, Miggiano GAD, Gasbarrini A, Mele MV. What is the healthy gut microbiota composition? A changing ecosystem across age, environment, diet. and diseases. Microorganisms. 2019;7:14.
Lloyd-Price J, Abu-Ali G, Huttenhower C. The healthy human microbiome. Genome Med. 2016;8:51.
Mariat D, Firmesse O, Levenez F, Guimarăes V, Sokol H, Doré J, Corthier G, Furet JP. The Firmicutes/Bacteroidetes ratio of the human microbiota changes with age. BMC Microbiol. 2009;9:123.
Jeffery IB, O’Toole PW, Öhman L, Claesson MJ, Deane J, Quigley EM, Simrén M. An irritable bowel syndrome subtype defined by species-specific alterations in faecal microbiota. Gut. 2012;61:997–1006.
Distrutti E, Monaldi L, Ricci P, Fiorucci S. Gut microbiota role in irritable bowel syndrome: New therapeutic strategies. World J Gastroenterol. 2016;22:2219–41.
Diviccaro S, Giatti S, Borgo F, Barcella M, Borghi E, Trejo JL, Garcia-Segura LM, Melcangi RC. Treatment of male rats with finasteride, an inhibitor of 5alpha-reductase enzyme, induces long-lasting effects on depressive-like behavior, hippocampal neurogenesis, neuroinflammation and gut microbiota composition. Psychoneuroendocrinology. 2019;99:206–15.
Wang L, Christophersen CT, Sorich MJ, Gerber JP, Angley MT, Conlon MA. Increased abundance of Sutterella spp. and Ruminococcus torques in feces of children with autism spectrum disorder. Mol Autism. 2013;4:42.
Williams BL, Hornig M, Parekh T, Lipkin WI. Application of novel PCR-based methods for detection, quantitation, and phylogenetic characterization of Sutterella species in intestinal biopsy samples from children with autism and gastrointestinal disturbances. MBio. 2012;3:pii: e00261–11.
Guo Q, Zheng Y, Shi J, Wang J, Li G, Li C. Immediate psychological distress in quarantined patients with COVID-19 and its association with peripheral inflammation: a mixed-method study. Brain Behav Immun. 2020;88:17–27.
Alpert O, Begun L, Garren P, Solhkhah R. Cytokine storm induced new onset depression in patients with COVID-19. A new look into the association between depression and cytokines -two case reports. Brain Behav Immun Health. 2020;9:100173.
Taquet M, Luciana S, Geddes JR, Harrison PJ. Bidirectional associations between COVID- 19 and psychiatric disorder: retrospective cohort studies of 62354 COVID-19 cases in the USA. Lancet Psychiatry. 2020. https://doi.org/10.1016/S2215-0366(20)30462-4 .
Mukaetova-Ladinska, EB, Kronenberg G. Psychological and neuropsychiatric implications of COVID-19. Eur Arch Psychiatry Clin Neurosci. 2020. https://doi.org/10.1007/s00406 - 020-01210-2
Mazza MG, De Lorenzo R, Conte C, Poletti S, Vai B, Bollettini I, Melloni EMT, Furlan R, Ciceri F, Rovere-Querini P, et al. Anxiety and depression in COVID-19 survivors: Role of inflammatory and clinical predictors. Brain Behav Immun. 2020;89:594–600.
Ahmed MZ, Ahmed O, Aibao Z, Hanbin S, Siyu L, Ahmad A. Epidemic of COVID-19 in China and associated psychological problems. Asian J Psychiatr. 2020;51:10209.
Steardo L, Steardo L, Verkhratsky A. Psychiatric face of COVID-19. Transl Psychiatry. 2020;10:261.
Coomes EA, Haghbayan H. Interleukin-6 in Covid-19: a systematic review and meta- analysis. Rev Med Virol. 2020;30:1–9.
Mojtabavi H, Saghazadeh A, Rezaei N. Interleukin-6 and severe COVID-19: a systematic review and meta-analysis. Eur Cytokine Netw. 2020;31:44–9.
Sun H, Guo P, Zhang L, Wang F. Serum Interleukin-6 Concentrations and the Severity of COVID-19 Pneumonia: A Retrospective Study at a Single Center in Bengbu City, Anhui Province, China. in January and February 2020. Med Sci Monit. 2020;26:e926941.
Gubernatorova EO, Gorshkova EA, Polinova AI, Drutskaya MS. IL-6: relevance for immunopathology of SARS-CoV-2. Cytokine Growth Factor Rev. 2020;53:13–24.
Liu B, Li M, Zhou Z, Guan X, Xiang Y. Can we use interleukin-6 (IL-6) blockade for coronavirus disease 2019 (COVID-19)-induced cytokine release syndrome (CRS)? J Autoimmun. 2020:102452.
Moore JB, June CH. Cytokine release syndrome in severe COVID- 19. Science. 2020;368:473–4.
Benedetti F, Mazza M, Cavalli G, Ciceri F, Dagna L, Rovere-Querini P. Can cytokine blocking prevent depression in COVID-19 survivors? J Neuroimmune Pharmacol. 2020:1–3.
Catanzaro M, Fagiani F, Racchi M, Corsini E, Govoni S, Lanni C. Immune response in COVID-19: addressing a pharmacological challenge by targeting pathways triggered by SARS-CoV-2. Sig Transduct Target Ther. 2020;5:84.
Download references
Acknowledgements
Not applicable
Financial support was solely provided by the authors.
Author information
Authors and affiliations.
Department of Clinical Pharmacy, Faculty of Pharmacy, Tehran Medical Sciences, Islamic Azad University, No. 99 Yakhchal Street, Shariati Avenue, Tehran, 1941933111, Iran
Elnaz Roohi & Farshad Hashemian
Université de Poitiers, Unité de recherche clinique intersectorielle Pierre Deniker du Centre Hospitalier Henri Laborit F-86022 France, Groupement De Recherche CNRS 3557, Poitiers, France
Nematollah Jaafari
You can also search for this author in PubMed Google Scholar
Contributions
ER contributed to the conception of the study, searched, screened, did the data extraction of the studies, drafted, and reviewed the manuscript. NJ contributed to the conception of the study, reviewed, and edited the manuscript. FH conceived the idea of the study, critically reviewed, and edited the manuscript. All authors read and approved the final version of the manuscript.
Corresponding author
Correspondence to Farshad Hashemian .
Ethics declarations
Ethics approval and consent to participate, consent for publication, competing interests.
The authors declare that they have no competing interests.
Additional information
Publisher’s note.
Springer Nature remains neutral with regard to jurisdictional claims in published maps and institutional affiliations.
Rights and permissions
Open Access This article is licensed under a Creative Commons Attribution 4.0 International License, which permits use, sharing, adaptation, distribution and reproduction in any medium or format, as long as you give appropriate credit to the original author(s) and the source, provide a link to the Creative Commons licence, and indicate if changes were made. The images or other third party material in this article are included in the article's Creative Commons licence, unless indicated otherwise in a credit line to the material. If material is not included in the article's Creative Commons licence and your intended use is not permitted by statutory regulation or exceeds the permitted use, you will need to obtain permission directly from the copyright holder. To view a copy of this licence, visit http://creativecommons.org/licenses/by/4.0/ . The Creative Commons Public Domain Dedication waiver ( http://creativecommons.org/publicdomain/zero/1.0/ ) applies to the data made available in this article, unless otherwise stated in a credit line to the data.
Reprints and permissions
About this article
Cite this article.
Roohi, E., Jaafari, N. & Hashemian, F. On inflammatory hypothesis of depression: what is the role of IL-6 in the middle of the chaos?. J Neuroinflammation 18 , 45 (2021). https://doi.org/10.1186/s12974-021-02100-7
Download citation
Received : 09 November 2020
Accepted : 02 February 2021
Published : 16 February 2021
DOI : https://doi.org/10.1186/s12974-021-02100-7
Share this article
Anyone you share the following link with will be able to read this content:
Sorry, a shareable link is not currently available for this article.
Provided by the Springer Nature SharedIt content-sharing initiative
- Neuroinflammation
- Interleukin-6
Journal of Neuroinflammation
ISSN: 1742-2094
- Submission enquiries: [email protected]
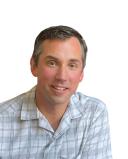
3 Signs of Hidden Depression
Despite the emotional tempest, signs of depression may be subtle..
Updated April 10, 2024 | Reviewed by Ray Parker
- What Is Depression?
- Find counselling to overcome depression
- Depression isn't always obvious.
- Noticing hidden signs can expedite evaluation and intervention.
- Sighing, frustration, and agitation can be revealing of underlying depression.
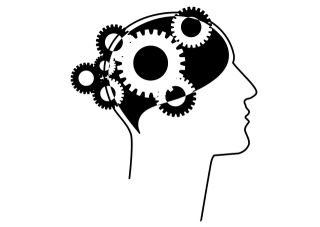
Depression is a ubiquitous term, and it seems most people have some idea, if not entirely accurate, of what it means. The symptom of sadness might come to mind for some, while others envision a suicidal gothic character. What lies between these extreme ends of the continuum—an isolated, fleeting symptom and an almost caricature representation—is vast. There are subtypes of depression, and even people suffering from the same subtype can present differently.
Further, some people are more internalized or externalized in their expression and possess greater or lesser abilities in managing. Jane, for instance, upon evaluation, might possess eight symptoms but seems OK because she works and cares for her family. Joe, on the other hand, might have five and be unable to care for himself. The severity of depression isn't necessarily weighed by several symptoms but by how impaired someone is by them. Regardless, given Joe’s symptoms are more visual, he might get help and live a higher quality of life while Jane silently suffers through life, or, worse, to a tragic ending.
With this in mind, it is important to pay attention to hidden signs of depression. There might be more to someone always sighing, frustrated, and agitated than meets the eye.
Unusual and Subtle Signs
Would it surprise you that depression can be hidden in plain sight, perhaps chameleonized as a "different issue?"
People arriving for sleep problems, anger , or infidelity may just be presenting the tip of the depression iceberg. As I wrote about in this earlier post, for instance, aggressive or thrill-seeking behaviors can be downstream effects of an internalized process. This is especially true for males/cultures where displaying emotional “weakness” is considered dishonorable.
Internalized emotional pressure, coupled with insomnia and poor diet , might escape as anger reactions. Cheating or indiscriminate sex may assuage one’s low self-image , providing a sense of desirability or thrill in the face of an otherwise flat internal landscape. Providers should always provide careful diagnostic assessments, as no symptom/behavior exists in a vacuum. Trying to address the anger or infidelity alone will only provide tenuous abatement at best, like clipping back a weed but leaving the root to grow it back.
More Complicated Dynamics
Anyone who has worked with angry people knows that “They’re just an angry person” isn’t likely. This is also the case with someone who sighs a lot, acts frustrated, and appears rattled. There’s an internal conflict being expressed, and often, a mood or personality complication behind it.
Behaviors are expressions of thoughts and feelings.
People who are depressed often present with sighing, frustration/irritability, and agitation, but if the package presents itself sans obvious depression, don’t write it off. Depression could be driving that demeanor, but the person is otherwise managing to conceal the foundational struggle. Take the case of Jessie (composite identity), who was referred by his employer to the employee assistance program for appearing increasingly discontent over recent months.
Jessie was never the life of the office, but he was dependable and pleasant enough. Insidiously, he had taken to exaggerated exhaling throughout the day, as if trying to externalize some burden so his day could go on. His work quality and productivity were not suffering, but he uncharacteristically became frustrated if there was a meeting or he was approached by colleagues, which disrupted his work. In meetings, it was hard not to notice his being fidgety or that he might roll his eyes and look about in an “I can’t believe this” gesture like his time was being wasted.
Jessie’s workload hadn’t changed, and there was no inter-collegiate problem present. However, his supervisor, Jenna, was concerned that his demeanor, despite his ongoing productivity, was placing a burden on the office milieu and referred him to the employee assistance program.
The Sigh-Depression Connection

Sighing alone isn’t necessarily a signal of negative emotionality ( Danvers et al ., 2021). We sigh every few minutes, as it is normal for respiratory function to inflate alveoli in the lungs (Li et al., 2016). However, when pronounced sighs are presented regularly with other observations, as Jenna noticed, maybe it’s time to check in with the person or oneself.
This is because we sigh more when stressed or emotional. Vlemincx et al. (2022) noted that “[Sighs reset] emotional states by facilitating emotional transitions.” Perhaps more frequent sighing helps people mired in negative emotions constantly reset to a manageable emotional state.
Agitation, of course, is not only a physical expression of one’s restless mental state but can serve as stimulation/preoccupation/distraction. It can also be an expression of frustration, which is not unusual in depressed people. This, of course, lends itself to sighing, too.
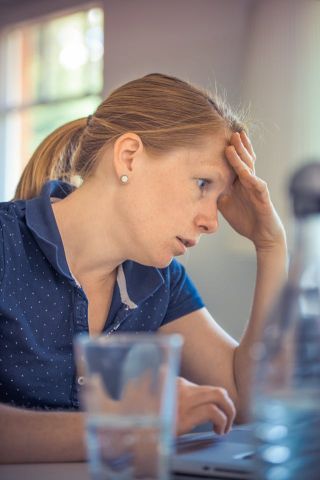
The inherent poor sleep and appetite of depression alone can cause irritation/agitation, but couple that with cognitive slowing and rumination of depression, and someone’s emotional experience could be akin to a pot with a lid on it beginning to boil; we don’t see the roil, but the shaking indicates something is up inside.
We all periodically roll our eyes, grunt, and/or hold our foreheads when frustrated, but when that fleeting state(s) becomes a trait, chronic internal turmoil likely keeps it afloat.
Clinical Implications
When encountering someone like Jessie, exploring their internal landscape is required for effective therapy :
After exchanging pleasantries, I said, “So, Jessie, we briefly spoke on the phone that you were referred because of seeming frustrated and on edge at work lately. What can you tell me about that?” Jessie went on to describe what Jenna did in the EAP referral.
“Seems like it’s become more noticeable over time," I replied. "I’m curious about what you can tell me about your experience as it was all building. Like, what changed? What has been on your mind in conjunction with all this?”
Though not directly answering the thought process question, Jessie reflected that for several months, he had been feeling “a grey mood” and could get irritable. He also slept more. It wasn’t the first time it happened. He remembered a similar event when his parents divorced when he was in his teens, 15 years earlier. Now, his mother had just divorced again and began “guilting” Jessie into doing things for her around the house. He set hard boundaries , engendering never-ending squabbling between him, his mother, and his siblings.
Jessie thought he kept any signs of bad moods hidden. Work was the most stimulating thing in his life at the moment, and he figured being occupied wouldn’t allow his bad mood to show through to colleagues. Work was an escape, but some of his inner world was escaping at work. Thankfully, it brought him to therapy, where he was able to work through his familial problems and not be left to stew in his emotions privately.
Robbins (2011) provides a good reminder of why it is important to keep vigilant for people like Jessie:
Because depression most immediately affects people’s inner mood and cognitive landscape, it is often “invisible” to outsiders. Thus, depression’s privacy can impede its early detection and thereby adequate treatment. These issues are compounded when the social stigma surrounding depression leads to patients intentionally hiding symptoms. Therefore, identifying observable, behavioral markers of depression is an important scientific endeavor with theoretical and clinical implications.
Danvers, A.F., Milek, A., Tackman, A.M., Kaplan, D.M., Robbins, M.L., Poslinelli, A., Moseley, S., Raison, C.L., Sbarra, D., & Mehl, M.R. (2021). Is frequent sighing an indicator of dispositional negative emotionality? A multi-sample, multi-measure naturalistic-observation study. Journal of Research in Personality , 90 , https://doi.org/10.1016/j.jrp.2020.104046
Li, P., Janczewski, W., Yackle, K., Kam, K., Pagliardini, S., Krasnow, M.A., & Feldman, J.A. (2016). The peptidergic control circuit for sighing. Nature, 530, 293–297 .
Robbins, M.L., Mehl, M.R., Holleran, S.E., & Kasle, S. (2011). Naturalistically observed sighing and depression in rheumatoid arthritis patients: a preliminary study. Health Psychology, 30 (1), 129-33. doi: 10.1037/a0021558. PMID: 21299301; PMCID: PMC3059549
Vlemincx, E., Severs, L., & Ramirez, J.M. (2022). The psychophysiology of the sigh: II: The sigh from the psychological perspective. Biological Psychology, 173 , https://doi.org/10.1016/j.biopsycho.2022.108386
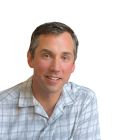
Anthony Smith, LMHC, has 23 years of experience that includes the roles of therapist, juvenile court evaluator, professor, and counseling supervisor.
- Find a Therapist
- Find a Treatment Center
- Find a Support Group
- International
- New Zealand
- South Africa
- Switzerland
- Asperger's
- Bipolar Disorder
- Chronic Pain
- Eating Disorders
- Passive Aggression
- Personality
- Goal Setting
- Positive Psychology
- Stopping Smoking
- Low Sexual Desire
- Relationships
- Child Development
- Therapy Center NEW
- Diagnosis Dictionary
- Types of Therapy
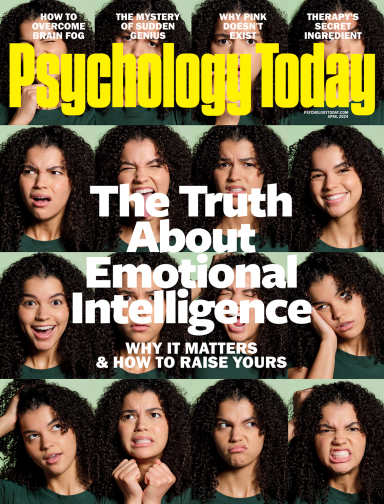
Understanding what emotional intelligence looks like and the steps needed to improve it could light a path to a more emotionally adept world.
- Coronavirus Disease 2019
- Affective Forecasting
- Neuroscience
Taylor Swift Tortured Poets Department 5 Stages of Grief Theory, Explained
By P. Claire Dodson
About a month out from Taylor Swift's 10th album The Tortured Poets Department , Swift has given her us her first real piece of album promotion since the Grammys announcement: a set of curated Apple Music playlists labeled with TTPD songs and related phrases that reflect the five stages of grief.
Over the past months, Swift has released album variants for TTPD in different sepia-toned colors with unique bonus tracks. Fans assembled them together and found a “Five Stages of Grief” chart that mirrors the colors, thus the theory was born that the album would be a literal interpretation of her breakup with Joe Alwyn and the ensuing grief she experienced.
The Apple Music intro implies, however, that the fan theory was what actually informed Swift's decision to compile and release these five playlists, not the other way around. “And when Swifties agree upon a theory, Taylor takes an interest—so naturally, she's responded by crafting a series of exclusive playlists, choosing songs of her own that fit each stage," the intro reads.
This content can also be viewed on the site it originates from.
Below, dive into each playlist and its tracklist before The Tortured Poets Department comes out April 19.
Denial: I Love You, It's Ruining My Life Songs
Taylor Swift's message: “This is a list of songs about getting so caught up in the idea of something that you have a hard time seeing the red flags possibly resulting in moments of denial and maybe a little bit of delusion.”
- Lavender Haze
- Snow On The Beach
- Sweet Nothing
- Cruel Summer
- Miss Americana & The Heartbreak Prince
- Wildest Dreams
- Treacherous
- Untouchable
- That’s When
Anger: You Don't Get to Tell Me About Sad Songs
Taylor Swift's message: “These songs all have one thing in common: I wrote them while feeling anger. Over the years I've learned that anger can manifest itself in a lot of different ways, but the healthiest way it can manifest itself in my life is when I can write a song about it.”
- Vigilante Shit
- High Infidelity
- Would’ve, Could’ve, Should’ve
- illicit affairs
- tolerate it
- Is It Over Now?
- I Knew You Were Trouble
- We Are Never Ever Getting Back Together
- The Last Time
- The Moment I Knew
- I Bet You Think About Me
- Better Than Revenge
- Tell Me Why
- You’re Not Sorry
- Forever & Always
- Mr. Perfectly Fine
Bargaining: Am I Allowed to Cry? Songs
Taylor Swift's message: “This playlist takes you through songs I've written when I was in the bargaining stage, times when you're trying to make deals with yourself or someone you care about, you're trying to make things better. You're often times feeling really desperate because oftentimes we have a gut intuition that tells us things are not going the way that we hope. Which makes us more desperate. Which makes us bargain more!"
- The Great War
- this is me trying
- Cornelia Street
- Death By A Thousand Cuts
- Soon You’ll Get Better
- I Wish You Would
- Say Don’t Go
- Come Back… Be Here
- The Story Of Us
- Come In With The Rain
- The Other Side Of The Door
- If This Was A Movie
Depression: Old Habits Die Screaming Songs
Taylor Swift's message: “We're going to be exploring the feelings of depression that often lace their way through my songs. In times like these, I'll write a song because I feel lonely or hopeless. And writing a song feels like the only way to process that intensity of an emotion. And while these things are really, really hard to go through, I often feel like when I'm either listening to songs or writing songs that deal with this intensity of loss and hopelessness — usually that's in the phase where I'm close to getting passed that feeling.”
- Bigger Than The Whole Sky
- Dear Reader
- You’re Losing Me
- my tears ricochet
- champagne problems
- coney island
- right where you left me
- Nothing New
- All Too Well
- Forever Winter
- e Were Happy
- Castles Crumbling
- White Horse
Acceptance: I Can Do It With a Broken Heart Songs
Taylor Swift's message: “We finally find acceptance and can start moving forward from loss or heartbreak. These songs represent making room for more good in your life, making that choice. Because a lot of the time when we lose things, we gain things too.”
By Liv McConnell
By Donya Momenian
By Njera Perkins
- You’re On Your Own, Kid
- Midnight Rain
- invisible string
- long story short
- it’s time to go
- I Forgot That You Existed
- Now That We Don’t Talk
- Begin Again
Thank you for visiting nature.com. You are using a browser version with limited support for CSS. To obtain the best experience, we recommend you use a more up to date browser (or turn off compatibility mode in Internet Explorer). In the meantime, to ensure continued support, we are displaying the site without styles and JavaScript.
- View all journals
- Explore content
- About the journal
- Publish with us
- Sign up for alerts
- Published: 05 April 2024
Genes associated with depression and coronary artery disease are enriched for cardiomyopathy and inflammatory phenotypes
- Kritika Singh ORCID: orcid.org/0000-0001-9872-7942 1 , 2 ,
- Hyunjoon Lee 3 , 4 , 5 na1 ,
- Julia M. Sealock 4 , 5 , 6 na1 ,
- Tyne Miller-Fleming 1 , 2 ,
- Peter Straub 1 , 2 ,
- Nancy J. Cox 1 , 2 ,
- Quinn S. Wells 7 ,
- Jordan W. Smoller 3 , 4 , 5 ,
- Emily C. Hodges 2 , 8 &
- Lea K. Davis ORCID: orcid.org/0000-0001-5143-2282 1 , 2 , 9 , 10 , 11
Nature Mental Health ( 2024 ) Cite this article
146 Accesses
112 Altmetric
Metrics details
- Gene regulation
Depression and coronary artery disease (CAD) are highly comorbid conditions. Approximately 40% of individuals who have one diagnosis will also develop the other within their lifetime. Despite the high prevalence of the comorbidity, the specific genes and pathways remain unknown. Here, by mapping known variants to genes, we identified genes, followed by pathways, that are associated with both depression and CAD. Next, we investigated the phenotypic consequences of the shared pathways in an electronic health record (EHR)-based setting. We identified 185 genes that were significantly associated with both depression and CAD and were enriched for inflammatory and cardiomyopathy phenotypes. We observed an increased rate of prevalent cardiomyopathy cases in individuals with comorbid depression–CAD compared with those with CAD alone in three large EHR datasets. The results of our study implicate genetically regulated inflammatory mechanisms in depression–CAD. Our results also raise the hypothesis that depression-associated CAD may be enriched for cardiomyopathy.
This is a preview of subscription content, access via your institution
Access options
Subscribe to this journal
Receive 12 digital issues and online access to articles
55,14 € per year
only 4,60 € per issue
Rent or buy this article
Prices vary by article type
Prices may be subject to local taxes which are calculated during checkout
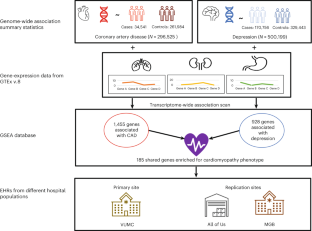
Similar content being viewed by others
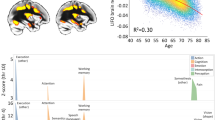
The effects of genetic and modifiable risk factors on brain regions vulnerable to ageing and disease
Jordi Manuello, Joosung Min, … Gwenaëlle Douaud
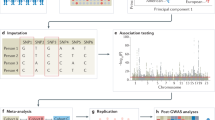
Genome-wide association studies
Emil Uffelmann, Qin Qin Huang, … Danielle Posthuma
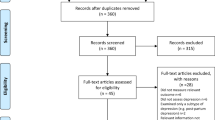
The serotonin theory of depression: a systematic umbrella review of the evidence
Joanna Moncrieff, Ruth E. Cooper, … Mark A. Horowitz
Data availability
Data are available from Vanderbilt University Medical Center with institutional restrictions that apply to the acquisition, use and dissemination of data. To request reasonable access to data for work conducted in a non-profit academic setting, please contact the Vanderbilt Institute for Clinical and Translational Research ([email protected]) and request an application to the Integrated Data Access and Services Core.
Code availability
The code for the analysis is available at https://bitbucket.org/davislabteam/depression-cad/src/master/ .
Kendler, K. S., Gardner, C. O., Fiske, A. & Gatz, M. Major depression and coronary artery disease in the Swedish twin registry: phenotypic, genetic, and environmental sources of comorbidity. Arch. Gen. Psychiatry 66 , 857–863 (2009).
Article PubMed PubMed Central Google Scholar
Glassman, A. H. Depression and cardiovascular comorbidity. Dialogues Clin. Neurosci. 9 , 9–17 (2007).
Sullivan, M. et al. Depression in coronary heart disease. What is the appropriate diagnostic threshold? Psychosomatics 40 , 286–292 (1999).
Article PubMed Google Scholar
O’Neil, A. et al. Depression is a risk factor for incident coronary heart disease in women: an 18-year longitudinal study. J. Affect. Disord. 196 , 117–124 (2016).
Weeke, A., Juel, K. & Vaeth, M. Cardiovascular death and manic-depressive psychosis. J. Affect. Disord. 13 , 287–292 (1987).
Dennis, J. et al. Genetic risk for major depressive disorder and loneliness in sex-specific associations with coronary artery disease. Mol. Psychiatry 26 , 4254–4264 (2021).
Mazereeuw, G. et al. Platelet activating factors in depression and coronary artery disease: a potential biomarker related to inflammatory mechanisms and neurodegeneration. Neurosci. Biobehav. Rev. 37 , 1611–1621 (2013).
McCaffery, J. M. et al. Common genetic vulnerability to depressive symptoms and coronary artery disease: a review and development of candidate genes related to inflammation and serotonin. Psychosom. Med. 68 , 187–200 (2006).
Upadhyay, R. K. Emerging risk biomarkers in cardiovascular diseases and disorders. J. Lipids 2015 , 971453 (2015).
Shimokawa, H., Aarhus, L. L. & Vanhoutte, P. M. Porcine coronary arteries with regenerated endothelium have a reduced endothelium-dependent responsiveness to aggregating platelets and serotonin. Circ. Res. 61 , 256–270 (1987).
Brydon, L., Magid, K. & Steptoe, A. Platelets, coronary heart disease, and stress. Brain Behav. Immun. 20 , 113–119 (2006).
Huang, M. et al. Longitudinal association of inflammation with depressive symptoms: a 7-year cross-lagged twin difference study. Brain Behav. Immun. 75 , 200–207 (2019).
Beydoun, M. A. et al. White blood cell inflammatory markers are associated with depressive symptoms in a longitudinal study of urban adults. Transl. Psychiatry 6 , e895 (2016).
Kim, J.-M. et al. Changes in pro-inflammatory cytokine levels and late-life depression: a two year population based longitudinal study. Psychoneuroendocrinology 90 , 85–91 (2018).
Bränn, E. et al. Inflammatory markers in late pregnancy in association with postpartum depression—a nested case-control study. Psychoneuroendocrinology 79 , 146–159 (2017).
Bremmer, M. A. et al. Inflammatory markers in late-life depression: results from a population-based study. J. Affect. Disord. 106 , 249–255 (2008).
Martínez-Cengotitabengoa, M. et al. Peripheral inflammatory parameters in late-life depression: a systematic review. Int. J. Mol. Sci. 17 , E2022 (2016).
Article Google Scholar
Gheysarzadeh, A. et al. Serum-based microRNA biomarkers for major depression: miR-16, miR-135a, and miR-1202. J. Res. Med. Sci. 23 , 69 (2018).
Schmidt, H. D., Shelton, R. C. & Duman, R. S. Functional biomarkers of depression: diagnosis, treatment, and pathophysiology. Neuropsychopharmacology 36 , 2375–2394 (2011).
Nurden, A. T. The biology of the platelet with special reference to inflammation, wound healing and immunity. Front. Biosci. 23 , 726–751 (2018).
Tsao, C.-W., Lin, Y.-S., Chen, C.-C., Bai, C.-H. & Wu, S.-R. Cytokines and serotonin transporter in patients with major depression. Prog. Neuropsychopharmacol. Biol. Psychiatry 30 , 899–905 (2006).
Pandey, G. N. et al. Hyperactive phosphoinositide signaling pathway in platelets of depressed patients: effect of desipramine treatment. Psychiatry Res. 105 , 23–32 (2001).
van der Harst, P. & Verweij, N. Identification of 64 novel genetic loci provides an expanded view on the genetic architecture of coronary artery disease. Circ. Res. 122 , 433–443 (2018).
Howard, D. M. et al. Genome-wide meta-analysis of depression identifies 102 independent variants and highlights the importance of the prefrontal brain regions. Nat. Neurosci. 22 , 343–352 (2019).
Barbeira, A. N. et al. Integrating predicted transcriptome from multiple tissues improves association detection. PLoS Genet. 15 , e1007889 (2019).
Sealock, J. M. et al. Use of the PsycheMERGE network to investigate the association between depression polygenic scores and white blood cell count. JAMA Psychiatry 78 , 1365 (2021).
Gidron, Y., Kupper, N., Kwaijtaal, M., Winter, J. & Denollet, J. Vagus–brain communication in atherosclerosis-related inflammation: a neuroimmunomodulation perspective of CAD. Atherosclerosis 195 , e1–e9 (2007).
Chan, K. L., Cathomas, F. & Russo, S. J. Central and peripheral inflammation link metabolic syndrome and major depressive disorder. Physiology 34 , 123–133 (2019).
Kempuraj, D. et al. Brain and peripheral atypical inflammatory mediators potentiate neuroinflammation and neurodegeneration. Front. Cell. Neurosci. 11 , 216 (2017).
Sun, Y., Koyama, Y. & Shimada, S. Inflammation from peripheral organs to the brain: how does systemic inflammation cause neuroinflammation? Front Aging Neurosci 14 , 903455 (2022).
Twig, G. et al. White blood cell count and the risk for coronary artery disease in young adults. PLoS ONE 7 , e47183 (2012).
Zebrack, J. S., Muhlestein, J. B., Horne, B. D. & Anderson, J. L. C-reactive protein and angiographic coronary artery disease: independent and additive predictors of risk in subjects with angina. J. Am. Coll. Cardiol. 39 , 632–637 (2002).
Lu, Q. et al. Intranuclear cardiac troponin I plays a functional role in regulating Atp2a2 expression in cardiomyocytes. Genes Dis. 15 , 1689–1700 (2021).
Google Scholar
Alimadadi, A., Munroe, P. B., Joe, B. & Cheng, X. Meta-analysis of dilated cardiomyopathy using cardiac RNA-seq transcriptomic datasets. Genes 11 , 60 (2020).
Nakajima, K. et al. Brain-specific heterozygous loss-of-function of ATP2A2, endoplasmic reticulum Ca 2+ pump responsible for Darier’s disease, causes behavioral abnormalities and a hyper-dopaminergic state. Hum. Mol. Genet. 30 , 1762–1772 (2021).
Coste de Bagneaux, P. et al. A homozygous missense variant in CACNB4 encoding the auxiliary calcium channel beta4 subunit causes a severe neurodevelopmental disorder and impairs channel and non-channel functions. PLoS Genet. 16 , e1008625 (2020).
Andrade, A. et al. Genetic associations between voltage-gated calcium channels and psychiatric disorders. Int. J. Mol. Sci. 20 , 3537 (2019).
Kanehisa, M. et al. KEGG for linking genomes to life and the environment. Nucleic Acids Res. 36 , D480–D484 (2007).
Xu, H. et al. A genome-wide association study of idiopathic dilated cardiomyopathy in African Americans. J. Pers. Med. 8 , 11 (2018).
Good, J.-M. et al. ACTN2 variant associated with a cardiac phenotype suggestive of left-dominant arrhythmogenic cardiomyopathy. HeartRhythm Case Rep. 6 , 15–19 (2020).
Haywood, N. J. et al. Hypertrophic cardiomyopathy mutations in the calponin-homology domain of ACTN2 affect actin binding and cardiomyocyte Z-disc incorporation. Biochem. J. 473 , 2485–2493 (2016).
Tiso, N. Identification of mutations in the cardiac ryanodine receptor gene in families affected with arrhythmogenic right ventricular cardiomyopathy type 2 (ARVD2). Hum. Mol. Genet. 10 , 189–194 (2001).
Patel, R. et al. Variants of trophic factors and expression of cardiac hypertrophy in patients with hypertrophic cardiomyopathy. J. Mol. Cell. Cardiol. 32 , 2369–2377 (2000).
Yadav, M. L., Bhasker, A. N., Kumar, A. & Mohapatra, B. Identification and characterization of genetic variants of TGFB1 in patients with congenital heart disease. Meta Gene 31 , 100987 (2022).
Bauer, R., MacGowan, G. A., Blain, A., Bushby, K. & Straub, V. Steroid treatment causes deterioration of myocardial function in the δ-sarcoglycan-deficient mouse model for dilated cardiomyopathy. Cardiovasc. Res. 79 , 652–661 (2008).
Rutschow, D. et al. S151A δ-sarcoglycan mutation causes a mild phenotype of cardiomyopathy in mice. Eur J Hum Genet 22 , 119–125 (2014).
Coughlin, S. S. Post-traumatic stress disorder and cardiovascular disease. Open Cardiovasc. Med. J. 5 , 164–170 (2011).
Polimanti, R. et al. Understanding the comorbidity between posttraumatic stress severity and coronary artery disease using genome-wide information and electronic health records. Mol. Psychiatry 27 , 3961–3969 (2022).
Stein, M. B. et al. Genome-wide association analyses of post-traumatic stress disorder and its symptom subdomains in the Million Veteran Program. Nat. Genet. 53 , 174–184 (2021).
Pathak, G. A. et al. Genetically regulated multi-omics study for symptom clusters of posttraumatic stress disorder highlights pleiotropy with hematologic and cardio-metabolic traits. Mol. Psychiatry 27 , 1394–1404 (2022).
Pathak, G. A. et al. Genetic liability to posttraumatic stress disorder symptoms and its association with cardiometabolic and respiratory outcomes. JAMA Psychiatry 81 , 34–44 (2024).
Lynall, M.-E. et al. Peripheral blood cell-stratified subgroups of inflamed depression. Biol. Psychiatry 88 , 185–196 (2020).
McNally, L., Bhagwagar, Z. & Hannestad, J. Inflammation, glutamate, and glia in depression: a literature review. CNS Spectr. 13 , 501–510 (2008).
Troubat, R. et al. Neuroinflammation and depression: a review. Eur J Neurosci 53 , 151–171 (2021).
Eitel, I. et al. Inflammation in takotsubo cardiomyopathy: insights from cardiovascular magnetic resonance imaging. Eur. Radiol. 20 , 422–431 (2010).
Adamo, L., Rocha-Resende, C., Prabhu, S. D. & Mann, D. L. Reappraising the role of inflammation in heart failure. Nat. Rev. Cardiol. 17 , 269–285 (2020).
Maisch, B., Ristic, A. D. & Pankuweit, S. Inflammatory cardiomyopathy and myocarditis. Herz 42 , 425–438 (2017).
Krejci, J., Mlejnek, D., Sochorova, D. & Nemec, P. Inflammatory cardiomyopathy: a current view on the pathophysiology, diagnosis, and treatment. BioMed Res. Int. 2016 , 4087632 (2016).
Tschöpe, C. et al. Myocarditis and inflammatory cardiomyopathy: current evidence and future directions. Nat. Rev. Cardiol. 18 , 169–193 (2021).
Eitel, I. et al. Clinical characteristics and cardiovascular magnetic resonance findings in stress (takotsubo) cardiomyopathy. JAMA 306 , 277–286 (2011).
PubMed Google Scholar
Scally, C. et al. Myocardial and systemic inflammation in acute stress-induced (takotsubo) cardiomyopathy. Circulation 139 , 1581–1592 (2019).
Rroku, A., Grahl, S., Landmesser, U. & Heidecker, B. A case report of myocardial inflammation in takotsubo syndrome. A chicken-or-the-egg phenomenon. Int. J. Cardiol. Heart Vasc. 39 , 100958 (2022).
PubMed PubMed Central Google Scholar
Lonsdale, J. et al. The Genotype-Tissue Expression (GTEx) project. Nat. Genet. 45 , 580–585 (2013).
Bowton, E. et al. Biobanks and electronic medical records: enabling cost-effective research. Sci. Transl. Med. 6 , 234cm3 (2014).
The All of Us Research Program Investigators. The ‘All of Us’ Research Program. N. Engl. J. Med. 381 , 668–676 (2019).
Download references
Acknowledgements
This research is supported by the American Heart Association Fellowship AHA827137 (K.S.), National Institute of Mental Health R56MH120736 (L.K.D.), National Institute of Mental Health R01 H118233 (L.K.D. and J.W.S.), National Institutes of Health 1F31MH124306-01A1 (J.M.S.), and National Institutes of Health 1R01HL140074 (Q.S.W.). The funders had no role in study design, data collection and analysis, decision to publish or preparation of the paper. The de-identified EHR used at VUMC was supported by the National Center for Research Resources, Grant UL1 RR024975-01, and is now at the National Center for Advancing Translational Sciences, Grant 2 UL1 TR000445-06. The content is solely the responsibility of the authors and does not necessarily represent the official views of the NIH. The dataset(s) used for the analyses described were obtained from Vanderbilt University Medical Center’s BioVU, which is supported by numerous sources: institutional funding, private agencies and federal grants. These include the NIH-funded Shared Instrumentation Grant S10RR025141, and CTSA grants UL1TR002243, UL1TR000445 and UL1RR024975. Genomic data are also supported by investigator-led projects that include U01HG004798, R01NS032830, RC2GM092618, P50GM115305, U01HG006378, U19HL065962 and R01HD074711, and additional funding sources listed at https://victr.vumc.org/biovu-funding/ . The All of Us Research Program is supported by grants through the National Institutes of Health, Office of the Director: Regional Medical Centers: 1 OT2 OD026549, 1 OT2 OD026554, 1 OT2 OD026557, 1 OT2 OD026556, 1 OT2 OD026550, 1 OT2 OD026552, 1 OT2 OD026553, 1 OT2 OD026548, 1 OT2 OD026551 and 1 OT2 OD026555; IAA#: AOD 16037; Federally Qualified Health Centers: HHSN 263201600085U; Data and Research Center: 5 U2C OD023196; Biobank: 1 U24 OD023121; The Participant Center: U24 OD023176; Participant Technology Systems Center: 1 U24 OD023163; Communications and Engagement: 3 OT2 OD023205 and 3 OT2 OD023206; and Community Partners: 1 OT2 OD025277, 3 OT2 OD025315, 1 OT2 OD025337 and 1 OT2 OD025276. In addition to the funded partners, the All of Us Research Program would not be possible without the contributions made by its participants.
Author information
These authors contributed equally: Hyunjoon Lee, Julia M. Sealock.
Authors and Affiliations
Division of Genetic Medicine, Department of Medicine, Vanderbilt University Medical Center, Nashville, TN, USA
Kritika Singh, Tyne Miller-Fleming, Peter Straub, Nancy J. Cox & Lea K. Davis
Vanderbilt Genetics Institute, Vanderbilt University Medical Center, Nashville, TN, USA
Kritika Singh, Tyne Miller-Fleming, Peter Straub, Nancy J. Cox, Emily C. Hodges & Lea K. Davis
Psychiatric and Neurodevelopmental Genetics Unit, Center for Genomic Medicine, Massachusetts General Hospital, Boston, MA, USA
Hyunjoon Lee & Jordan W. Smoller
Center for Precision Psychiatry, Department of Psychiatry, Massachusetts General Hospital, Boston, MA, USA
Hyunjoon Lee, Julia M. Sealock & Jordan W. Smoller
Stanley Center for Psychiatric Research, Broad Institute of Harvard and MIT, Cambridge, MA, USA
Analytical and Translational Genetics Unit, Massachusetts General Hospital, Boston, MA, USA
Julia M. Sealock
Division of Cardiovascular Medicine, Department of Medicine, Vanderbilt University Medical Center, Nashville, TN, USA
Quinn S. Wells
Department of Biochemistry, Vanderbilt University School of Medicine, Nashville, TN, USA
Emily C. Hodges
Department of Molecular Physiology and Biophysics, Vanderbilt University Medical Center, Nashville, TN, USA
Lea K. Davis
Department of Psychiatry and Behavioral Sciences, Vanderbilt University Medical Center, Nashville, TN, USA
Department of Medicine and Biomedical Informatics, Vanderbilt University Medical Center, Nashville, TN, USA
You can also search for this author in PubMed Google Scholar
Contributions
K.S. and L.K.D. conceptualized and designed the work. K.S., J.M.S., T.M.-F., P.S. and H.L. implemented the computational procedures and performed data analysis. N.J.C., J.W.S., Q.S.W. and E.C.H. provided important clinical and intellectual insights. All authors read, edited and approved the final paper.
Corresponding author
Correspondence to Lea K. Davis .
Ethics declarations
Competing interests.
J.W.S. is a member of the Scientific Advisory Board of Sensorium Therapeutics (with equity) and has received an honorarium for an internal seminar Tempus Labs. He is a principal investigator of a collaborative study of the genetics of depression and bipolar disorder sponsored by 23andMe for which 23andMe provides analysis time as in-kind support but no payments. The other authors declare no competing interests.
Peer review
Peer review information.
Nature Mental Health thanks Janitza Montalvo-Ortiz and the other, anonymous reviewer(s) for their contribution to the peer review of this work.
Additional information
Publisher’s note Springer Nature remains neutral with regard to jurisdictional claims in published maps and institutional affiliations.
Supplementary information
Supplementary information.
Supplementary methods and results.
Reporting Summary
Supplementary tables.
Supplementary Tables 1–10.
Rights and permissions
Springer Nature or its licensor (e.g. a society or other partner) holds exclusive rights to this article under a publishing agreement with the author(s) or other rightsholder(s); author self-archiving of the accepted manuscript version of this article is solely governed by the terms of such publishing agreement and applicable law.
Reprints and permissions
About this article
Cite this article.
Singh, K., Lee, H., Sealock, J.M. et al. Genes associated with depression and coronary artery disease are enriched for cardiomyopathy and inflammatory phenotypes. Nat. Mental Health (2024). https://doi.org/10.1038/s44220-024-00219-z
Download citation
Received : 14 January 2023
Accepted : 22 February 2024
Published : 05 April 2024
DOI : https://doi.org/10.1038/s44220-024-00219-z
Share this article
Anyone you share the following link with will be able to read this content:
Sorry, a shareable link is not currently available for this article.
Provided by the Springer Nature SharedIt content-sharing initiative
Quick links
- Explore articles by subject
- Guide to authors
- Editorial policies
Sign up for the Nature Briefing newsletter — what matters in science, free to your inbox daily.

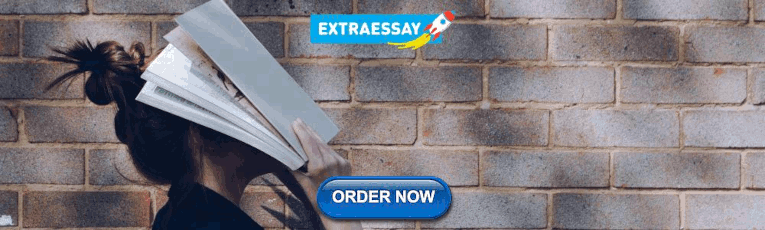
IMAGES
VIDEO
COMMENTS
This hypothesis is relevant because most antidepressant drugs either prevent or reverse the damage caused by increased cortisol release, over-excitation, and inflammation [70,71]. Additionally, ECT is one possible adjunctive therapy for depression that canprevent or reverse the effects of cortisol and overexcitation . The factor linking ...
Numerous investigations have demonstrated that 5-HT is intimately related to the pathophysiological process of major depression. The 5-HT hypothesis primarily asserts that a decrease in the 5-HT ...
The serotonin hypothesis of depression is still influential. We aimed to synthesise and evaluate evidence on whether depression is associated with lowered serotonin concentration or activity in a ...
1. Introduction. Depression as described by the World Health Organization (WHO) is a mood disorder characterized by specific symptoms including sadness, loss of interest, anhedonia (loss of pleasure), lack of appetite, feelings of guilt, low self-esteem or self-worth, sleep disturbance, feelings of tiredness, and poor concentration [1].Individuals suffering from depression describe varying ...
Abstract. Hypotheses about the pathophysiology of depression have evolved over time. This chapter covers the most important findings in this regard. First, the classical monoamine hypothesis posited that depression is caused by an alteration in levels of one or more of the monoamines: serotonin, norepinephrine, and dopamine.
The notion that clinical depression might be caused by deficient activity of the brain neurotransmitter, serotonin (5-hydroxytryptamine (5-HT)) is over 50 years old. This theory seems to have been first proposed in 1967 by the British psychiatrist, Alec Coppen, though in his review, Coppen mentions several other lines of aetiological research ...
The monoamine hypothesis of depression postulates a deficiency in serotonin or norepinephrine neurotransmission in the brain. Monoaminergic neurotransmission is mediated by serotonin (5 ...
The hypothesis that major depression represents a deficiency of norepinephrine (NE) at critical synapses in the central nervous system has been extremely influential since the mid-1960s.
The Basics. Depression was recognized by Hippocrates (ca. 460-377 B.C.), Galen (ca. 129-199 A.D.), and Ishaq Ibn Imran (10th century A.D.), and these physicians' early clinical descriptions well mirror those of today, including a profound loss of the capacity to feel pleasure, severe dysphoria (despondency), and a loss of will ().These symptoms are similar to those of severe bereavement ...
Although "the serotonin hypothesis of depression is still influential," Moncrieff and coauthors noted, citing widely adopted textbooks published as recently as 2020 and surveys indicating that ...
The neurotrophic hypothesis of depression [1] proposes that major depressive disorder (MDD) is caused, at least partly, by impaired neurotrophic support. Neurotrophic factors (also known as neurotrophins) are a family of closely related proteins which regulate the survival, development, and function of neurons in both the central and peripheral ...
Antidepressants remain an effective treatment for depression, even without the "chemical imbalance" explanation A recent umbrella review of evidence for the serotonin theory of depression1 was widely reported in UK media as showing that depression is not caused by low levels of serotonin or a "chemical imbalance" and therefore casting doubt on the use of selective serotonin reuptake ...
Psychodynamic Theory. Cognitive Explanation of Depression. Learned Helplessness. Humanist Approach. Depression is a mood disorder that prevents individuals from leading a normal life at work, socially, or within their family. Seligman (1973) referred to depression as the 'common cold' of psychiatry because of its frequency of diagnosis.
ABSTRACT. The separation distress hypothesis of depression, formulated in 2009, offered an affective neuroscience perspective on our most common and costly mental health condition, as an alternative to molecular reductionism in psychiatry. Our hypothesis constituted a neurobiological extension of classic work on depression as intrinsically ...
The main areas of research do not provide support for the most well-known neurochemical hypothesis, the serotonin theory of depression—the idea that depression is caused by low levels or low ...
Many patients with major depressive disorder (MDD) are reported to have higher levels of multiple inflammatory cytokines including interleukin 6 (IL-6). Recent studies both pre-clinical and clinical have advocated for the functional role of IL-6 in development of MDD and suggested a great potential for targeting this cytokine to open new avenues in pharmacotherapy of depression. The purpose of ...
Freud believed that depression is an exaggerated feeling of guilt and self-blame that results from early life experiences and interactions. He viewed the mind as a dynamic of three parts: id, superego, and ego. He also viewed depression as a reaction to difficult situations that is turned inward. Learn more about his theory, modern research, and how to apply it to your own life.
Noticing hidden signs can expedite evaluation and intervention. Sighing, frustration, and agitation can be revealing of underlying depression. Depression is a ubiquitous term, and it seems most ...
Parents, siblings and children of people with treatment-resistant depression are nine times more likely to develop the same disorder, according to a new study. CNN values your feedback 1.
The'opioid hypothesis of depression' and the 'glutamate hypothesis of depression' are being promoted alongside efforts to market opioids and ketamine (which acts on glutamate ...
I Forgot That You Existed. Daylight. This Love. Clean. Now That We Don't Talk. Begin Again. Innocent. Breathe. Taylor Swift was inspired by a popular Tortured Poets Department album fan theory ...
The clinical co-occurrence of depression and cardiomyopathy, taken together with findings from the TWAS implicating immune genes, raises the hypothesis that chronic low-grade inflammation may ...