Land Degradation
Land degradation is a process in which the value of the biophysical environment is influenced by a variety of human-induced activities that operate on the land. It is defined as any modification or disturbance to the land that is either detrimental or unwanted. Natural hazards are not considered a cause; nonetheless, human actions can have an indirect impact on phenomena such as floods and bushfires. Because of the consequences for agricultural production, the environment, and food security, this is seen as an essential problem for the twenty-first century. It is believed that up to 4% of the world’s agricultural land has been severely damaged.
The soil provides a growing medium for a variety of plant types. It also serves as a home for a variety of animals and microbes that contribute significantly to the ecological balance. When the composition of the soil is contaminated as a result of the disposal of hazardous waste or the usage of chemicals, the organisms that rely on the soil for nutrition suffer. The environmental impact of soil contamination or land pollution is frequently overlooked in comparison to other types of pollution.
According to the Intergovernmental Panel on Climate Change’s Special Report on Climate Change and Land: “Human-induced deterioration affects around a fifth of the Earth’s ice-free land area (medium confidence). Soil erosion from agricultural fields is currently estimated to be 10 to 20 times (no-tillage) to more than 100 times (conventional tillage) greater than the rate of soil formation (medium confidence).”
Land degradation is caused by a variety of factors, including harsh weather, notably drought. It is also caused by human actions that contaminate or impair soil and land utility quality. It has a negative impact on food production, livelihoods, as well as the production and delivery of other ecosystem goods and services. Desertification is a type of land degradation in which productive land deteriorates into desert.
One of the biggest causes of soil degradation is the disposal of garbage that cannot be degraded naturally, such as various types of plastic materials, Styrofoam, metal scraps, and so on. These wastes remain in the soil and have an impact on its fertility. Irresponsible dumping of biodegradable or organic waste can also cause contamination and render land areas useless owing to filth.
According to the United Nations, around 30% of the world’s land is deteriorated, and over 3.2 billion people live in these degraded areas. Every year, around 12 million hectares of productive land — nearly the size of Greece – gets degraded. This occurs as a result of individuals using the land without protecting it. The United Nations Sustainable Development Goal 15 has a target to restore degraded land and soil and achieve a land degradation-neutral world by 2030.
Another factor that has contributed to the destruction of many locations is mining. Deep mining creates deep and open shafts, rendering the ground unfit for cultivation or living. The subsurface empty spaces caused by mining can result in the formation of various sinkholes, which can be harmful in many cases. The continual drilling also loosens the soil and promotes erosion.
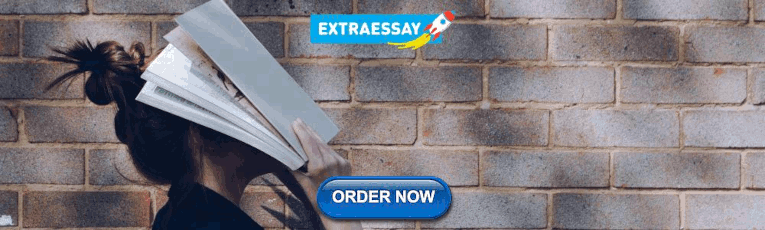
A Greenland Glacier That Was Once Stable Is Now Rapidly Disappearing
Marine plastic pollution, scientists explain how a historic ban on cfcs gave the world a fighting chance to combat global warming, traffic noise, good manufacturing practices, monetary inflation, annual report 2006 of grameenphone limited, foreign exchange controls, annual report 2012-2013 of wipro limited, annual report 2015-2016 of state bank of mysore, latest post, cathodic modification, anodic protection (ap), new maps assist decision-makers in considering albedo when planting trees, experts fear that climate change will exacerbate the spread of infectious diseases, curbside pickup enhances organic waste composting and decreases methane emissions, key concepts of electromagnetic induction.
- Assessing knowledge Monitoring assessment Second global assessment Nexus assessment Transformative change assessment Business and biodiversity assessment Biodiversity and climate change Invasive alien species assessment Sustainable use of wild species assessment Values assessment Global assessment Land degradation and restoration assessment Land degradation and restoration assessment events Land degradation and restoration assessment experts Regional assessments Scenarios and models assessment Pollination assessment Guide on the production of assessments
- Building capacity
- Strengthening the knowledge foundations
- Supporting policy
- Communicating and engaging
- Improving the effectiveness of the platform
Assessment Report on Land Degradation and Restoration
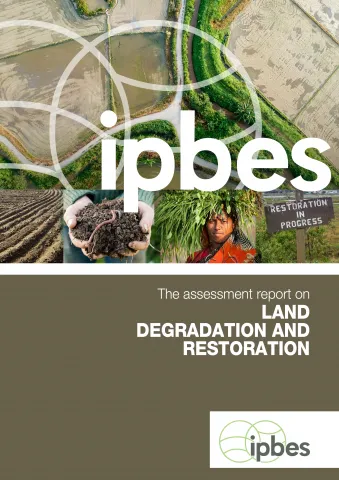
The assessment of land degradation and restoration covers the global status of and trends in land degradation, by region and land cover type; the effect of degradation on biodiversity values, ecosystem services and human well-being; and the state of knowledge, by region and land cover type, of ecosystem restoration extent and options. The assessment aims to enhance the knowledge base for policies for addressing land degradation, desertification and the restoration of degraded land.
In January 2015, at IPBES 3, the Plenary approved the launch of the land degradation and restoration assessment together with an agreed scope.
The Plenary approved the Summary for Policymakers and accepted the chapters of the Assessment at its 6 th session in March 2018 in Medellin, Colombia (IPBES 6). IPBES would like to acknowledge and thank all of the experts who contributed to the Assessment .
The IPBES Assessment Report on Land Degradation and Restoration is composed of:
- A Summary for Policymakers (SPM); and
- A set of 8 chapters.
Please see below to access the Assessment Report and related documents.
Editors : Luca Montanarella, Robert Scholes, Anastasia Brainich
Members of the management committee who provided guidance for the production of this assessment: Günay Erpul, Yi Huang, Marie Roué, Leng Guan Saw (Multidisciplinary Expert Panel), Rashad Zabid Oglu Allahverdiyev, Ivar Andreas Baste, Fundisile Goodman Mketeni (Bureau).
Suggested citation: IPBES (2018): The IPBES assessment report on land degradation and restoration. Montanarella, L., Scholes, R., and Brainich, A. (eds.). Secretariat of the Intergovernmental Science-Policy Platform on Biodiversity and Ecosystem Services, Bonn, Germany. 744 pages. https://doi.org/10.5281/zenodo.3237392
Summary for policymakers
Summary for policymakers (spm) - land degradation and restoration (laid out), summary for policymakers (spm) - land degradation and restoration (plain text), the assessment report on land degradation and restoration (full report, including spm and chapters; laid out), supplementary material, comments first review, comment/first review/ldr/chapter 1, comment/first review/ldr/chapter 2, comment/first review/ldr/chapter 3, comment/first review/ldr/chapter 4, comment/first review/ldr/chapter 5, comment/first review/ldr/chapter 6, comment/first review/ldr/chapter 7, comment/first review/ldr/chapter 8, comments second review, comments/second review/ldr/chapter 1, comments/second review/ldr/chapter 2, comments/second review/ldr/chapter 3, comments/second review/ldr/chapter 4, comments/second review/ldr/chapter 5, comments/second review/ldr/chapter 6, comments/second review/ldr/chapter 7, comments/second review/ldr/chapter 8, comments/second review/ldr/spm.
Global Assessment of Land Degradation and Improvement (GLADA)
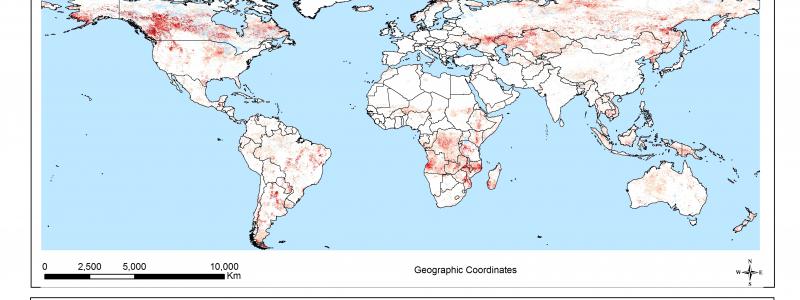
Ever-increasing demands on the land from economic development, burgeoning cities, and growing rural populations are driving unprecedented land-use change. In turn, land-use change is driving land degradation: soil erosion, nutrient depletion, salinity, water scarcity, pollution, disruption of biological cycles, and loss of biodiversity. This is a global issue (UNCED 1992, UNEP 2006), yet there is no authoritative measure of land degradation or its counterpoint – land improvement. The only harmonized assessment, the Global Assessment of Human-induced Soil Degradation is a map of perceptions on the type and degree of degradation. Dating from 1991, it is now out-of-date.
There is a pressing need for an up-to-date, quantitative and reproducible assessment to support policy development for food and water security, environmental integrity, and national strategies for economic development and resource conservation. In response, within the GEF-UNEP-FAO program Land Degradation in Drylands, the Global Assessment of Land Degradation and Improvement (GLADA) will identify:
- The status and trends of land degradation, and
- Hotspots suffering extreme constraints or at severe risk and, also, areas where degradation has been arrested or reversed.
Biomass is an integrated measure of biological productivity. Its deviance from the local norm may be taken as a measure of land degradation or improvement. Changes in biomass may be measured by remote sensing of the normalized difference vegetation index (NDVI - the difference between reflected near-infrared and visible wavebands divided by the sum of these two wavebands). NDVI has a strong, linear relationship with the fraction of photosynthetically active radiation absorbed by the plant; it is strongly correlated with vegetation cover and above-ground net primary productivity. Norms may be established by stratifying the land area according to climate, soils, and terrain, and land use/vegetation; deviance may then be calculated regionally and combined globally to allow universal comparisons.
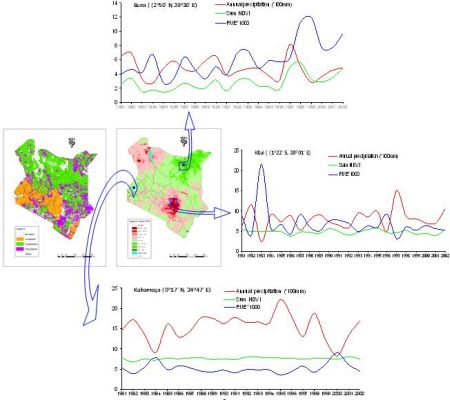
GLADA pilot study for Kenya
FAO has commissioned ISRIC to undertake pilot studies to:
- Elaborate the general approach to map global land degradation by analysis of the GIMMS dataset of 8km-resolution NDVI data;
- Analyze other global databases, in particular, the Soil and Terrain Database (SOTER), in support of the general NDVI methodology;
- Illustrate the approach developed under 1 and 2 with case studies in Kenya ( PDF ) and North China ( PDF ) ;
- Compare the results for Kenya with results from a high-resolution land cover change study produced by the Global Land Cover Network.
The general approach developed for GLADA involves a sequence of analyses to identify land degradation hotspots using remotely sensed data and existing datasets: first using simple NDVI indicators such as mean annual sum NDVI (surrogate for mean annual biomass production) and the trend of biomass production; secondly integration of biomass and climatic data (rain-use efficiency); thirdly, stratification of the landscape using land cover and soil and terrain data to enable a more localized analysis of the NDVI data. Most of the development work has gone into algorithms that enable these screening analyses to be undertaken automatically. At the next stage, the identified hotspots will be characterized manually, using 30m-resolution Landsat data, to identify the probable kinds of land degradation, preliminary to field examination by national teams within the wider LADA program.
Publications
Bai ZG, Jong de R, van Lynden GWJ 2010. An update of GLADA – Global assessment of land degradation and improvement. ISRIC report 2010/08, ISRIC – World Soil Information, Wageningen, 58p PDF
Bai ZG, Dent DL, Olsson L and Schaepman ME 2008. Global Assessment of Land Degradation and Improvement. 1 Identification by remote sensing. Report 2008/01(GLADA Report 5), ISRIC – World Soil Information, Wageningen, 70p PDF
Bai ZG and Dent DL 2007. Land degradation and improvement in Tunisia. 1: Identification by remote sensing. Report 2007/08 (GLADA Report 1f), ISRIC – World Soil Information, Wageningen, 47p PDF
Bai ZG and Dent DL 2007. Land degradation and improvement in Senegal. 1: Identification by remote sensing. Report 2007/07 (GLADA Report 1e), ISRIC – World Soil Information, Wageningen, 48p PDF
Bai ZG and Dent DL 2007. Land degradation and improvement in China. 1: Identification by remote sensing. Report 2007/06 (GLADA Report 1d), ISRIC – World Soil Information, Wageningen, 52p PDF
Bai ZG and Dent DL 2007. Land degradation and improvement in Argentina. 1: Identification by remote sensing. Report 2007/05 (GLADA Report 1c), ISRIC – World Soil Information, Wageningen, 50p PDF
Bai ZG and Dent DL 2007. Land degradation and improvement in Cuba. 1: Identification by remote sensing. Report 2007/04 (GLADA Report 1b), ISRIC – World Soil Information, Wageningen, 48p PDF
Bai ZG and Dent DL 2007. Land degradation and improvement in South Africa. 1: Identification by remote sensing. Report 2007/03 (GLADA Report 1a), ISRIC – World Soil Information, Wageningen, 54p
Other reports and news on the Land Degradation Assessment (LADA) Project are available from the FAO LADA website
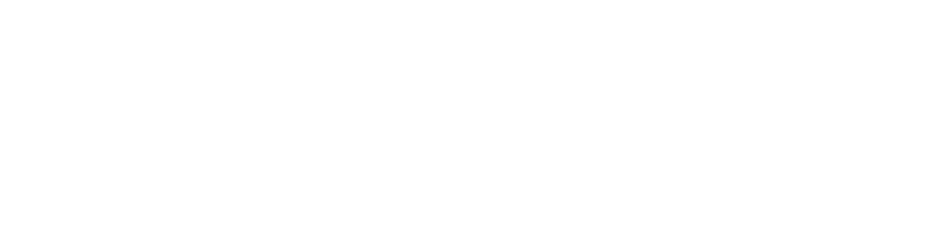
Approaches to assess land degradation risk: a synthesis

- Annegret H. Thieken , Annegret H. Thieken University of Potsdam, Institute of Earth and Environmental Science, Potsdam, Germany
- Eva Nora Paton Eva Nora Paton Technical University (TU) Berlin, Institute of Ecology, Berlin, Germany
> × Details
The following is the established format for referencing this article:
https://doi.org/10.5751/ES-13951-280153
- Introduction: Land Degradation in the Context of Disaster Risk Research
- Results: Identification of Key Issues
- Discussion: What is the Dilemma of Current Land Degradation Risk Assessment Approaches?
- Conclusions
- Responses to This Article
- Author Contributions
- Acknowledgments
- Data Availability
- Literature Cited
Article Metrics
Copyright and permissions.
Land degradation adversely affects the well-being of approximately 3.2 billion people worldwide and results in a loss of about 10% of annual gross domestic product. Degradation dynamics are often creeping and non-linear, hence the adverse consequences are often not immediately perceived. In consequence, methods for assessing future risks of land degradation and risk reduction strategies are trailing far behind those that have been developed within disaster risk research for natural hazards that appear as shocks, such as floods or earthquakes. Therefore, the objective of this paper is to analyze existing land degradation risk assessment approaches to assess what is hindering a link to land management strategies. The synthesis presented here reveals that while approaches to calculate land degradation risk have evolved to capture ever more processes and factors involved in land degradation, no consistent conceptual framework for land degradation risk has been developed to the present day. Key identified short-comings are that risk terminology is not consistent across and within studies and that there is often no distinction between a degradation status assessment and an assessment of future risk. Damage is rarely explicitly considered or quantified, and in the majority of studies there is no clear distinction between processes and drivers, hazard and vulnerability. Finally, novel conceptual ideas integrating the risk framework developed within disaster risk research are proposed to stimulate debate and facilitate the development of effective risk reduction measures for land degradation.
INTRODUCTION: LAND DEGRADATION IN THE CONTEXT OF DISASTER RISK RESEARCH
Land degradation represents one of the most urgent challenges for humanity, because it leads to an often permanent reduction of the land’s biological productivity, yield reduction, or loss, causing economic loss and hunger (Olsson et al. 2019). It adversely affects the well-being of approximately 3.2 billion people worldwide and results in a loss of about 10% of annual gross domestic product (GDP; IPBES 2018). Furthermore, the Millennium Ecosystem Assessment (MA 2005) revealed that around the year 2000 60% of the examined ecosystem services were being degraded or used unsustainably. Nevertheless, the development of effective disaster risk reduction (DRR) strategies in the context of policy and decision making has mostly focused on sudden-onset hazards that appear as shocks, such as floods or earthquakes, while slow-onset events, such as land degradation and drought, have been rarely considered within the conventional disaster risk framework (Matthewman 2015, Zaidi 2018, Hsu 2019, Staupe-Delgado 2019). This synthesis sets out to analyze the discrepancies in risk concepts used in the academic literature on land degradation risk assessment and explores ways to bridge the gap between land degradation research and DRR frameworks.
Over the last 30 years, definitions of land degradation have changed considerably (see Box 1), and according to the Intergovernmental Panel on Climate Change (IPCC) there is still “ no single method by which land degradation can be measured objectively and consistently over large areas because it is such a complex and value-laden concept ” (Olsson et al. 2019:348). In consequence, there is a wide variation in estimates of the global costs of land degradation, which range from US$17.58 billion to as high as US$9.4 trillion (Nkonya et al. 2016), and its current global extent and severity are still not well quantified (Olsson et al. 2019).
FAO 1979: “ Land degradation is a process which lowers the current or potential capability of soils to produce. ” (FAO 1979, as cited in Nachtergaele et al. 2010:73)
UNCCD 1994: “ Desertification means land degradation in arid, semi-arid, and dry sub-humid areas resulting from various factors, including climatic variations and human activities. ” (UNCCD 1994:4)
“ Land degradation in arid, semi-arid, and dry sub-humid areas is a reduction or loss of the biological or economic productivity and integrity of rainfed cropland, irrigated cropland, or range, pasture, forest, and woodlands resulting from land uses or from a process or combination of processes, including processes arising from human activities and habitation patterns, such as (i) soil erosion caused by wind and/or water; (ii) deterioration of the physical, chemical, biological, or economic properties of soil; and (iii) long-term loss of natural vegetation. ” (UNCCD 1994:5)
LADA 2011: “ Land degradation is the reduction in the capacity of the land to provide ecosystem goods and services, over a period of time, for its beneficiaries. ” (LADA 2011:7)
IPCC 2019: “ Land degradation is a negative trend in land condition, caused by direct or indirect human-induced processes including anthropogenic climate change, expressed as long-term reduction or loss of at least one of the following: biological productivity, ecological integrity or value to humans. ” (Olsson et al. 2019:349)
In this paper, we follow the most recent definition proposed by IPCC (Olsson et al. 2019) that defines land degradation as a negative trend in land condition, caused by human-induced processes, expressed as long-term reduction or loss of biological productivity, ecological integrity or value to humans. The processes of land degradation are diverse, encompassing, among others, soil erosion and salinization of the soil, as well as a persistent loss of natural vegetation cover or loss of biodiversity (IPBES 2018, Olsson et al. 2019). All of these processes act on individual temporal and spatial scales, and the dominating processes vary locally. This makes general assessments of land degradation on large scales difficult. The same holds for drivers of land degradation, mainly climate variability (e.g., the frequency of drought or extreme rainfall events) and human activities such as unsustainable agriculture. Finally, processes and drivers are often intermingled (Olsson et al. 2019). The result is that it is often not clear what should be measured, the current state of the ecosystem (e.g., vegetation coverage), the rate of the processes involved (e.g., erosion rate), or the local intensity of the drivers (e.g., intensity of land use, climatic drivers, etc.).
As opposed to sudden-onset hazards that appear as shocks, such as floods or earthquakes, land degradation processes can continue unnoticed for decades before adverse consequences appear (e.g., loss of ecological integrity, biological productivity, or ecosystem services). Research has shown that land degradation dynamics are often non-linear and may unfold gradually as well as suddenly (Turnbull et al. 2008, Karssenberg et al. 2017). Ecological tipping points, points where the system rapidly reorganizes into an alternative system state (Kéfi et al. 2014, Scheffer et al. 2015), can result in rapid cascading changes in ecosystems, even if external drivers change only gradually with time (Scheffer et al. 2001, Scheffer and Carpenter 2003, Turnbull et al. 2008). These non-linear dynamics make ecological regime shifts very hard to predict (Hastings and Wysham 2010), and the consequences may even become apparent only after a regime shift has already happened (Scheffer 1990). Once the ecosystem has been shifted to a degraded functional state, the process is often very difficult and costly to reverse because of positive stabilizing feedback mechanisms (Tomaselli 1977, Scheffer 1990, Rietkerk and van de Koppel 1997, Mäler 2000, Roques et al. 2001).
In consequence, the high damage potential of land degradation has long been concealed and land degradation has rarely been considered as a natural hazard in the context of disaster risk research. The adverse impacts of degradation can be intermingled with the effects of extreme hydrometeorological extreme events, which have often been perceived as the imminent threat in the past, particularly droughts (in this paper we refer to droughts as “ the naturally occurring phenomenon that exists when precipitation has been significantly below normal recorded levels, causing serious hydrological imbalances that adversely affect land resource production systems, ” UNCCD 1994:5). One prominent example is the prolonged drought in the Sahel region of sub-Saharan Africa in the 1970s and early 1980s that led to the death of over 200,000 people and widespread devastation of rural agriculture including the loss of millions of livestock (UNCCD 2020), providing the background for holding the first United Nations Conference on Desertification (UNCCD) in 1977 (Dregne 2002). The conference officially introduced the term “ desertification ” as a synonym for land degradation in drylands (Dregne 2002, UNCCD 2020). At first, drought was identified as the main factor for the human Sahel crises in the 1970s. Yet, it soon became clear that drought was not the underlying factor, but that prior land management was a crucial factor in the extent of the damage to the land (Dregne 2002), i.e., it appeared to be a man-made loss in the system’s ecological resilience that had made the land so vulnerable to droughts. In ecology, resilience is defined as a system’s ability to maintain its structural integrity and persist without being pushed into another stable state under the influence of a disturbance (Holling 1973, 1996, Scheffer et al. 2015). The notion that land degradation plays a crucial role in the land’s vulnerability to drought and that drought is often the mere trigger for human catastrophes, not the root cause, has since been a recurring notion in the literature on drought risk (see, e.g., Plate and Merz 2001, Felgentreff and Glade 2007, IPCC 2012, UNDRR 2019).
The relation between land degradation, drought, and human catastrophes exemplifies the finding that when both natural and human vulnerabilities occur at the same time and place, disasters can result (Alcántara-Ayala 2002). In the Sendai Framework of Disaster Risk Reduction 2015–2030 (UNISDR 2015), the United Nations established international procedures for disaster risk reduction (DRR). DRR aims at preventing new and reducing existing disaster risks and increasing resilience (UNDRR 2022). In order to promote a common understanding of DRR concepts and assist the implementation of DRR efforts of authorities, practitioners, and the public, the United Nations Office for Disaster Risk Reduction (UNDRR, formerly UNISDR) developed a coherent terminology related to DRR (Table 1) that is used as a guiding framework in this study.
Understanding risk as a concomitance of hazard, exposure, and vulnerability emphasizes that risk can be managed by modifying its components, i.e., reducing vulnerability or exposure to the hazard (Cardona 2004) and thus helps to identify effective entry points for DRR. It is based on Kaplan and Garrick (1981:13), who suggested that “ risk is probability and consequence. ” Disaster risk can be derived spatially from a combination of a hazard and a vulnerability map (Plate and Merz 2001); or, it can be represented in the form of risk curves, where the annual frequency of events with a certain magnitude is plotted over their potential overall damage. The unit of risk is then given as the average economic damage or by human loss indicators (loss of lives, people suffering from adverse health effects, etc.) expected per year (Plate and Merz 2001, Merz et al. 2009).
Natural hazards are natural processes or phenomena “ that may cause loss of life, injury or other health impacts, property damage, social and economic disruption or environmental degradation ” (UNDRR 2022). In disaster risk research the hazard is usually expressed as the probability of occurrence of an event with a certain magnitude at a given location during a specified period of time. The extent to which hazards result in actual damage depends on both the spatial and temporal exposure of the elements at risk and their vulnerability. Vulnerability represents the predisposition of elements that makes them susceptible to the impact of hazards (Cardona 2004, UNDRR 2022) and is expressed in the form of potential damage affecting people, property, or natural capital (Plate and Merz 2001). It depends on the susceptibility (also called sensitivity) as well as the resilience of the affected elements.
Risk assessment forms an important part of DRR, because it constitutes the basis for decision makers’ evaluation of risks. It is described as “ a qualitative or quantitative approach to determine the nature and extent of disaster risk by analyzing potential hazards and evaluating existing conditions of exposure and vulnerability that together could harm people, property, services, livelihoods and the environment on which they depend ” (UNDRR 2022).
The objective of this synthesis is to analyze the discrepancies in existing land degradation risk assessment approaches, with regard to the disaster risk concept of the UNDRR as summarized in Table 1. By this we want to identify key elements that hinder a link between land degradation risk assessment and land management. We further investigate how the underlying risk concept affects the way results can be linked to decision making in land management. A systematically chosen sample of the scientific literature was analyzed with regard to the underlying risk concept and its components (hazard, exposure, vulnerability), the perspective on land degradation, its processes and drivers, as well as its temporal component. These themes are covered in separate subsections of the results. The studies addressing land degradation with a risk concept that is in line with UNDRR’s definitions are presented separately at the end of the results section. In the discussion, eight key issues currently hindering a link between land degradation risk assessment and land management are identified based on the preceding literature analysis. Finally, based on identified discrepancies, ideas toward a comprehensive land degradation risk assessment are proposed as a way forward, by incorporating the concepts of ecological resilience and disaster risk research.
A systematic literature search was conducted in the scientific database of the Web of Science ( http://apps.webofknowledge.com ). Originally drafted as part of a PhD thesis (von Keyserlingk 2021; date of original literature search: 25 January 2017), the search was extended for this paper to cover the time period from 1900 to 22 December 2021 and the text revised. A set of title search criteria was chosen to identify studies explicitly aiming at a land degradation risk assessment: “ (‘land degradation’ OR desertification) AND risk AND (analys* OR assess*). ” The keyword “ desertification ” was included in addition to “ land degradation, ” because by definition it refers to land degradation in drylands (UNCCD 1994), and the two terms are often used synonymously. The additional terms “ analys* ” or “ assess* ” were included, for if only the term “ risk ” was used, a manual examination of the abstracts revealed that the vast majority of results were not related to a systematic risk assessment approach. Rather, the term “ risk ” was used in its colloquial meaning. For the same reason, a title search was chosen over a topic search. Only original research articles available in the English language were included. The systematic search resulted in a database of 33 studies covering 30 years (1992–2021, Appendix 1, Table A1). This selection of studies is not intended to be exhaustive of all research performed on the topic, but should present an objectively chosen sample as a basis for the analysis.
The focus of the analysis was to understand in which context the term “ risk ” was used in each study and how the underlying risk concept affects the possibilities for potentially linking results of the risk analysis with decision making in land management. Hence, the first step was to assess whether risk was used with an (explicit or implicit) underlying risk concept or whether it was used in its colloquial meaning. In a second step, the risk concept was analyzed with regard to the concept of disaster risk used by the UNDRR (Table 1). Studies were loosely grouped according to their understanding of risk (Table A1) to promote a joint discussion of studies on selected thematic aspects related to the underlying risk concept. In the results section, studies are presented with regard to the following questions: In which context was the term “ risk ” used? What was the underlying risk concept and terminology including the risk components of hazard and vulnerability? Was there a clear distinction between risk, hazard, and vulnerability, between cause and effect? How was the temporal component of land degradation risk addressed? Which studies understood risk as a concomitance of hazard and vulnerability (i.e., similar to the disaster risk concept used by UNDRR) and how was this implemented in the risk analysis?
RESULTS: IDENTIFICATION OF KEY ISSUES
Ambiguity of the term “ risk ”.
The process of searching for studies that conceptually address risk assessment related to land degradation or desertification first of all revealed that the term “ risk ” was frequently used with the intention of alerting the reader to the seriousness of the problem of land degradation and to the extent of the associated damage to human livelihoods, without any linkage to a conceptual risk framework. Hence, it proved challenging to develop a search strategy by which studies that at least mostly address risk within a conceptual context could be efficiently extracted. The title search criteria described above proved sufficient to meet this goal. Still, seven of the 33 studies (Rubio and Bochet 1998, Taguas et al. 2013, Vorovencii 2015, 2016, 2017, Abdallah and Massoud 2018, Türkes et al. 2020; see Table A1) do not provide any explanation of their conceptual understanding of risk, even though all of them refer to risk assessment for degradation or desertification in the title. The study by Rubio and Bochet (1998) aims at discussing and selecting suitable indicators for a desertification risk assessment. With the expression “ desertification risk assessment, ” they refer to an “ evaluation system of desertification. ” In Taguas et al. (2013), the aim stated in the title, a land degradation risk assessment, does not connect to the rest of the article, which is concerned with the calculation of annual soil erosivity from precipitation records. Although this approach takes into account that degradation is a process rather than a fixed state, it does not aim at estimating future risks. Within the text, the terms “ risk ” and “ soil erosion risk ” are used with the intention to highlight the potential negative consequences arising from soil erosion. The two studies by Vorovencii (2015, 2016) explicitly aim at a risk assessment. Again, risk assessment is interpreted as an assessment of desertification status (here, based on satellite-derived vegetation greenness, landscape pattern, and surface albedo), which was repeated for several points in time. Additionally, in the study from 2016, the convergence to degraded land is assessed over time; yet, this is not included in their calculation of risk classes. The term “ risk ” is used very frequently in both studies within various phrases connected to desertification (e.g., “ the risk of exposure to desertification ” [Vorovencii 2015:7], “ exposed to desertification risk ” [Vorovencii 2015:9], “ measures for fighting against the land degradation risk ” [Vorovencii 2016:15], but also to drivers of desertification (e.g., “ the risk of destructuring ” or “ the risk of overgrazing ” [in Vorovencii 2016:11,13]). The different ways in which “ risk ” is used reveals that it is not related to any conceptual framework. Further, there is no clear distinction between hazard and risk, and the hazard is not clearly identified. For example, the hazard could be the desertification processes or it could be drivers of desertification, such as overgrazing. The damaging consequences of desertification are clearly stressed as translating to, among others, a reduction or loss of the biological potential of the land (Vorovencii 2015). In summary, the studies that do not relate the term “ risk ” to any conceptual framework use it with a colloquial meaning with the intention of stressing the threatening nature of land degradation. All of them appear to confuse a risk assessment with an assessment of the land degradation status or of certain degradation processes.
Risk concepts and terminology: risk, hazard and vulnerability
Twenty-five of the analyzed studies use the term “ risk ” within a conceptual, risk-related framework (see Table A1). However, most of them do not provide an explicit definition of risk (exceptions are: Ubugunov et al. 2011, Ali and Abdel Kawy 2013, Hai et al. 2013, Wang et al. 2015, Akbari et al. 2016, Salunkhe et al. 2018, Martínez-Valderrama et al. 2020, Masoudi et al. 2021a, 2021b; see Table A1). Three studies (Ubugunov et al. 2011, Wang et al. 2015, Akbari et al. 2016; see Table A1) can be related to the risk concept of UNDRR used in disaster risk research and are discussed separately at the end of the results section. For the other 22 studies, a conceptual framework could be either explicitly or implicitly discerned (see Table A1 for an overview of the respective risk concepts). The analysis revealed that the inherent risk concepts and terminology were not consistent among and within studies. Four of the studies (Masoudi and Jokar 2018, Abu Seif and El-Khashab 2019, Masoudi et al. 2021a, 2021b; see Table A1) do not distinguish between “ hazard ” and “ risk. ” In Masoudi et al. (2021a) and in Masoudi et al. (2021b) risk is defined as the probability of hazard occurrence. In the title of Abu Seif and El-Khashab (2019) a “ desertification risk assessment ” is stated, yet later the “ desertification hazard of sand dunes ” (p. 359) is assessed. Even more frequently, “ risk ” is used synonymously with “ vulnerability ” (namely in: Salvati et al. 2008, Salvati and Zitti 2009, Ladisa et al. 2012, Becerril-Piña et al. 2015, Salvati et al. 2015, Tombolini et al. 2016, Uzuner and Dengiz 2020). For example, Salvati et al. (2008) use desertification risk synonymously with “ environmental vulnerability. ” In Salvati et al. (2015) spatial vulnerability patterns are used to assess desertification risk. Furthermore, the term “ vulnerability ” is recurrently used in substitution with “ susceptibility ” or “ sensitivity ” (e.g., in Ladisa et al. 2012). In Vieira et al. (2021) “ desertification risk ” is used synonymously with “ desertification susceptibility. ” Garg and Harrison (1992) as well as Ali and Abdel Kawy (2013) use “ erosion risk ” synonymously with “ susceptibility to erosion. ”
This ambiguity in terminology indicates that no consistent framework including the different risk components exists in the academic land degradation literature and that they are not clearly distinguished from each other. The confusion of risk with vulnerability is frequently associated with an analysis implemented with a geographic information system (GIS), such as the Environmental Sensitive Areas (ESA) framework (based on the EU-funded MEDALUS [Mediterranean Desertification and Land Use] project) - one of the most common approaches to determine desertification risk (Salvati and Zitti 2009). It has been applied by eight studies (Salvati et al. 2008, Salvati and Zitti 2009, Ladisa et al. 2012, Tombolini et al. 2016, Karamesouti et al. 2018, Salunkhe et al. 2018, Pishyar et al. 2020, Uzuner and Dengiz 2020). In this methodology, an Environmental Sensitive Area Index (ESAI) is calculated based on four thematic indicators representing soil quality, climate, vegetation, and management practices / land use (Ladisa et al. 2012, Tombolini et al. 2016). Sometimes, additional socioeconomic factors such as human pressure or demographic trends are added (namely in: Salvati et al. 2008, Ladisa et al. 2012, Salunkhe et al. 2018). The ESAI is calculated as the geometric mean of these indices. The results are generally presented in spatially explicit risk maps identifying different classes of areas “ sensitive to desertification ” ranging from “ non-affected ” to “ critical ” (e.g., in Ladisa et al. 2012). What appears striking is that the ESAI is explicitly called a “ risk index, ” e.g., by Salvati et al. (2008), and is commonly used to perform “ desertification risk assessment, ” while at the same time it is widely recognized as a measure of vulnerability to desertification (Salvati and Zitti 2009, Tombolini et al. 2016). The naming of the index itself and its categories refer to environmentally sensitive areas. This highlights the confusion of the terms risk, vulnerability, and sensitivity.
Different perspectives on land degradation: how to distinguish between processes and drivers, cause and effect, hazard and vulnerability
What remains generally unclear is what the land is vulnerable to. Not only is there usually no distinction between hazard and vulnerability, but also the perspectives on land degradation vary among studies. Whereas some studies focus on soil-related processes (e.g., Garg and Harrison 1992, Ali and Abdel Kawy 2013, Taguas et al. 2013), the majority of studies (e.g., Ibáñez et al. 2008, Becerril-Piña et al. 2015, Wang et al. 2015, Weinzierl et al. 2016) follow the more modern, holistic view on land degradation, including different components of the human-environment system (see an overview of land degradation definitions in Box 1). Based on this perspective, it has become common practice to look at the bulk impact of land degradation processes rather than assessing individual processes (Ibáñez et al. 2008). According to Salvati and Zitti (2009:960), the ESA approach does match this requirement exactly, because it “ does not focus on a specific process of land degradation ... but quantifies the synergic impact of different factors potentially leading to land degradation. ” Other risk indices follow a similar approach. For example, Santini et al. (2010) combine vegetation, soil properties, soil erosion, seawater intrusion, and grazing models to calculate a Desertification Risk Index. Similarly, Karavitis et al. (2020) calculate a desertification risk index (DRI) based on a combination of indicators related to the physical environment, land management, and socioeconomic factors. Weinzierl et al. (2016) calculate an Integrated Degradation Risk Index based on topography, land cover, soil, land use, demography, infrastructure, and climate. Although approaches such as this one indeed account in detail for many factors associated with land degradation, they create another problem: When all factors are merged within one index, it becomes impossible to differentiate between drivers (e.g., climatic variation and land use) and processes (e.g., soil erosion rate, seawater intrusion, etc.) of land degradation. Additionally, the bio-physical susceptibility (e.g., topography, soil and vegetation properties) of the land itself cannot be disentangled. Last, expected damage cannot be quantified, for it is intermingled within the risk index.
Among the analyzed literature, several studies effectively distinguishing between cause and effect in land degradation risk were identified. Those that can be related to the risk concept used by UNDRR are discussed separately in the last part of the results section. Other approaches were found in Ali and Abdel Kawy (2013), Hai et al. (2013), Ibáñez et al. (2008), and Martínez-Valderrama et al. (2020), and are discussed below. The modeling study by Ibáñez et al. (2008) uses system stability analysis to identify long-term land use conditions leading to desertification. Assuming constant climate and economic scenarios, they focus on the human-resource system. Resource exploitation is founded on economic decisions. Simulations revealed that high-profit scenarios are responsible for the expansion of desertification. The model clearly distinguishes between the drivers (here: human activity) and the result (here: loss in provision of resources). Thereby, Ibáñez et al. (2008) indirectly interpret human overexploitation of resources as the hazard, and the loss in provision of resources, i.e., land degradation, as the potential damage (i.e., the vulnerability). Another interesting, yet very different approach that clearly differentiates between cause and effect was found in Hai et al. (2013). Here, a probabilistic understanding of risk is expressed and risk is defined as “ the chance of something happening that will have impacts on objectives ” (Hai et al. 2013:1546). The authors used a semi-qualitative approach focusing on cause-effect relationships that are arranged in a risk assessment matrix ( “ Leopold Matrix ” after Leopold et al. 1971). Individual socioeconomic activities that are potentially affected by desertification, such as rice or cotton cultivation, raising livestock, or water supply, were collected together with a set of environmental and socioeconomic factors associated with desertification (such as land use, topography, rainfall, population density, etc.) potentially affecting those activities. Risk scores were calculated for each target activity based on categories representing the likelihood of occurrence of particular causes (i.e., “ hazards, ” ranging from “ very unlikely ” to “ almost certain ” ) in combination with ranks of consequences (i.e., “ vulnerability, ” ranging from “ no impact ” to “ catastrophic ” ). The risk calculation is hence based on a combination of likelihood and consequence, and as such shows striking parallels to quantitative risk assessment. However, the assignment of the categories for likelihood as well as for consequences is not based on quantitative data. Rather, stakeholder knowledge and risk perceptions were used as the main input to the risk matrix following a qualitative social sciences approach. Risk scores are not used to quantify expected damage, but to determine which environmental and socioeconomic factors pose the highest threat to socio-economic activities. As such, the approach of using a risk matrix can still assist management decision making, even though it lacks objectivity and precision. Finally, the study by Ali and Abdel Kawy (2013) indirectly estimates the degree to which land use causes soil degradation. Soil degradation risk is calculated by multiplying soil, topographic, and climate ratings in GIS, resulting in a risk map. They differentiate between the “ degradation risk, ” which they also call the “ susceptibility to degradation ” (Ali and Abdel Kawy 2013:2774), and the “ degradation hazard, ” here meaning the actual presence of degradation processes (salinization, sodification, water logging). The difference between these two is attributed to human impact (positive or negative); i.e., land that has a low degradation risk, but is actually strongly degraded indicates land mismanagement, while land with a high degradation risk, but a low degradation status is managed beneficially. Thereby, inappropriate land use practices can be identified.
These three examples illustrate that a differentiation between cause (hazard) and effect (vulnerability) directly creates a link to decision making in land management. However, the diversity of approaches and lack of a shared risk concept and terminology hamper coherent land degradation risk assessment.
Temporal component of land degradation risk
Several studies (namely: Salvati and Zitti 2009, Santini et al. 2010, Becerril-Piña et al. 2015, Salvati et al. 2015, Tombolini et al. 2016, Vorovencii 2016, 2017, Weinzierl et al. 2016, Karamesouti et al. 2018, Masoudi et al. 2021a, 2021b) consider temporal trends in their analysis, thereby accounting for the dynamic nature of land degradation, as well as for the potential of future risk. Santini et al. (2010:395) point out in their introduction that “ an area mildly degraded subject to an intense and rapid desertification process is more at risk when compared to an area at higher degradation level but stable over time. ” They account for this by including temporal degradation trends in their risk calculation. Yet, in the end, the seriously degraded areas are assigned to the “ very high risk class, ” whereas critical areas at a “ precarious equilibrium between the natural environment and human activities ” are assigned to the “ high risk class ” (Santini et al. 2010:406). This classification appears contradictory to their statement in the introduction. An integration of static and temporal components within one risk index (as in Santini et al. 2010 or in Weinzierl et al. 2016) makes it impossible to disentangle future risk from the present degradation status. Another approach to account for the dynamic nature of risk is a spatiotemporal convergence analysis, as performed by Salvati and Zitti (2009), Tombolini et al. (2016), and Vorovencii (2016). Here, spatial convergence of land degradation status or vulnerability is calculated over time to estimate future degradation trends, which is interpreted as risk. This approach indicates that risk is seen as something dynamic, different from a degradation status assessment. Becerril-Piña et al. (2015) base their risk assessment purely on the temporal component of risk: They did not include any static factors such as terrain or soil properties, but analyzed changes in temporal trends of land degradation factors (vegetation, climate, and anthropogenic). Ibáñez et al. (2008) and Martínez-Valderrama et al. (2020) treat risk of desertification as the likelihood that an undesirable system state is reached in the long term, thereby clearly addressing desertification risk as something potentially happening in the future. Martínez-Valderrama et al. (2020) used a state and transition model of social-ecological systems and assessed risk based on the percentage of simulations in which the final stable state was undesirable (key resources exhausted or critical thresholds crossed). Three studies (Masoudi and Jokar 2018, Masoudi et al. 2021b, 2021a) clearly distinguish between the present degradation status (termed “ actual risk ” in Masoudi and Jokar 2018) and “ potential risk ” of degradation in the future. Furthermore, they introduce a statistical picture of risk by assigning probability levels to those areas at potential risk of becoming further degraded in the future.
In conclusion, the incorporation of a temporal component in risk estimation is a necessary step that promotes, but does not necessarily lead to, a clear distinction between land degradation status and future risk. Accounting for a probabilistic nature of risk, as has been done in more recent studies, promotes such a distinction, thereby facilitating a link to land management. In contrast, assessing risk based on changes in temporal degradation trends alone disregards other elements of land degradation risk, such as vulnerability, thereby hindering a link to land management. The scenario-based approach applied in state and transition models is a very valuable step toward understanding the effects of land management decisions on land degradation risk over the long term.
Land degradation risk assessment approaches related to the disaster risk concept of UNDRR
The approaches attempting a land degradation risk assessment that can be linked to the disaster risk concept of UNDRR (namely: Ubugunov et al. 2011, Wang et al. 2015, Akbari et al. 2016; see Table A1) have in common that all of them include an explicit risk definition that clearly distinguishes hazard from vulnerability. The hazard is seen as consisting of anthropogenic factors, such as agricultural activities or livestock grazing, as well as natural factors, including climatic effects such as precipitation, evaporation, and wind. Some properties of the land itself, such as vegetation coverage (in Wang et al. 2015) are included here too, but they also form a part of the vulnerability (e.g., soil properties in Wang et al. 2015). In Ubugunov et al. (2011) the properties of the land itself are described as endogenous hazards, whereas climate and human activities are described as exogenous hazards. At the same time, Ubugunov et al. (2011) repeatedly use the phrase “ desertification hazard, ” indicating that the occurrence of desertification processes themselves is seen as the hazard. In line with this, they consider areas exposed to certain desertification processes (water erosion, deflation, salinization) in their risk analysis (see Table 1 in Ubugunov et al. 2011:182). In Akbari et al. (2016), desertification severity is referred to as representing the hazard. However, the assessment of desertification severity is also referred to as the “ calculation of the risk ” (Ubugunov et al. 2011:370). This shows that the terms “ risk ” and “ hazard ” are not clearly distinguished from each other.
With the vulnerability term, potential damage is considered. In the three studies discussed here, vulnerability refers to a potential loss in ecosystem productivity resulting in economic losses, i.e., by considering the fraction of agricultural land affected by land degradation as in Ubugunov et al. (2011), or by including the ratios of the agricultural population and agricultural outputs to the gross domestic product (GDP) as in Wang et al. (2015). Additionally, the vulnerability term includes ecosystem properties, such as vegetation cover (Akbari et al. 2016), and soil properties (Wang et al. 2015), which make land vulnerable to degradation processes. This shows that vulnerability is approached on two levels: first, on the socioeconomic level, and second, on the ecosystem level. In Wang et al. (2015) restorability is considered separately, yet purely in a socioeconomic context (i.e., referring to the level of education and to a variety of measures for disaster prevention such as rehabilitation measures). In Akbari et al. (2016) it does not become clear what the vulnerability term consists of. Vulnerability is described as a “ function of stability (resilience) and instability (vulnerability), ” as well as a “ function of resilience and sensitivity of the elements at risk ” (Akbari et al. 2016:369). The first definition appears to be inconsistent, for it defines vulnerability as a function of vulnerability itself. A vulnerability value is assigned based on social-ecological conditions, but also on the presence of risk itself, for they write that “ elements that are at higher risk classes will be more vulnerable ” (Akbari et al. 2016:369, see also their Table 4). This appears to be circular reasoning. With “ risk class ” they seem to refer to the desertification severity, again confusing risk and hazard.
The dynamic component of land degradation risk is approached in contrasting ways. Akbari et al. (2016) do not consider a dynamic risk component at all. In Ubugunov et al. (2011), the inherently probabilistic nature of both hazards and vulnerability is explicitly acknowledged: risk is quantified as expected annual damage. Yet, at the same time, the authors understand desertification as a permanent hazard existing “ during the entire length of time of its action ” (Ubugunov et al. 2011:180) and keep the probability term constant over time. Thus, although the inclusion of a probabilistic term within a risk model is a novelty of this study, its implementation has obvious shortcomings. In Wang et al. (2015:1712) the hazard term is described as the “ variation degree of natural disaster and human factors which caused the desertification disaster. ” Even though no probabilistic term is explicitly included, risk is seen as something dynamic, depending on the variation of natural disasters. Yet, this dynamic idea is not coherently followed up on in their analysis (except that some of the input datasets they used are continuous, e.g., meteorological data, normalized difference vegetation index with 16-day resolution). Interestingly, in contrast to Ubugunov et al. (2011), they describe desertification as a “ typical disaster risk event ” (Wang et al. 2015:1703), which suggests a discrete event in time.
In Ubugunov et al. (2011) the results of their risk analysis are presented quantitatively, giving direct estimates for the different administrative districts of the Republic of Buryatia: in affected hectares/year for the physical desertification risk, in rubles/year for the economic risk, and in the number of affected persons/year. It is the only study where expected damages are quantitatively assessed. Both in Akbari et al. (2016) and in Wang et al. (2015) risk is presented in the form of an index, with risk categories ranging from low to very high risk. Separate maps for the different components of risk (hazard, elements at risk, vulnerability [+ restorability in Wang et al. 2015]), as well as final risk maps are produced. The study by Wang et al. (2015) is unique in clearly presenting which factors were included in which risk component (see Fig. 4 in Wang et al. 2015:1713).
The approaches applying a natural disaster risk assessment to land degradation show that even though they share a similar risk concept, the terminology is inconsistently used within and among studies. Vulnerability and hazard are still difficult to disentangle. Only in Wang et al. (2015) is it clearly discernible what factors the different components of risk are composed of. None of the studies present a comprehensive solution for capturing the dynamic nature of land degradation adequately to allow projections of future risks. This highlights the difficulty of including a probabilistic approach in land degradation risk assessment. Often, the analysis falls short of the concept.
DISCUSSION: WHAT IS THE DILEMMA OF CURRENT LAND DEGRADATION RISK ASSESSMENT APPROACHES?
Land degradation describes a variety of processes that lead to a reduction of the land’s biological productivity, integrity, or value to humans (Olsson et al. 2019). Hence, the definition itself contains an explicit inclusion of ecological and socioeconomic damage resulting from these processes (see Box 1). This provides a direct link to the vulnerability concept as an expression of potential damage resulting from a hazard, as it is used in the context of disaster risk research. Curiously, however, to the present day no consistent framework for a quantitative assessment of land degradation risk appears to have been developed: The synthesis presented here has revealed that while approaches to calculate land degradation risk have evolved to include and capture ever more processes and factors involved in land degradation and risk assessment in detail, the development of a coherent conceptual risk framework is trailing behind. At the same time, an urgent need to derive “ measures for fighting against the land degradation risk ” (Vorovencii 2016:15) appears to be generally well-recognized. A quantification of that risk, however, is hampered by an overall discrepancy in what this risk contains. The analysis of 33 studies dealing with land degradation risk assessment approaches revealed that not even a consensus exists on what is perceived as the hazard. Altogether, eight key issues could be identified, hampering the development of a stringent and coherent risk assessment for land degradation and a link to risk-informed decision making:
- The term “ risk ” is frequently used in its colloquial meaning, with the intention of alerting and emphasizing the seriousness of the problem of land degradation and the extent of potential damage to human livelihoods. This emphasizes that the perception of land degradation as a threat to humanity is there, even though it is not connected to a formal risk concept.
- Risk assessment is often confused with a degradation or desertification status or process assessment (i.e., monitoring of land degradation). This confusion hinders a link to the different phases of disaster risk management (i.e., prevention, preparedness, response, and recovery; UNISDR (2015)) and a projection of future risks. This is an important barrier to linking risk analysis to land management options and interventions.
- Risk terminology is not consistently used among and within studies. The terms risk, hazard, vulnerability, and susceptibility are often confused. Only a minority of studies (Ubugunov et al. 2011, Hai et al. 2013, Wang et al. 2015, Akbari et al. 2016, Salunkhe et al. 2018, Martínez-Valderrama et al. 2020) include an explicit risk definition. Even if a risk theory exists, it is not consistent between studies and often the analysis falls short of the concept.
- Risk assessment and vulnerability assessment are often confused and used synonymously. Vulnerability is usually not linked to potential damage to the people (exceptions are: Ubugunov et al. 2011, Hai et al. 2013, Wang et al. 2015), but rather used as a descriptor for the land’s likelihood of becoming degraded. This likelihood is assessed based on a combination of environmental susceptibility to degradation and the present land degradation status (plus past trends), as well as on climatic and human drivers. The confusion of risk and vulnerability assessment makes it impossible to differentiate between present land degradation status and future risks.
- Potential damage (i.e., average annual economic or ecological damage, number of injured persons, or loss of lives) is mentioned frequently as a motivation or to raise attention to the urgency of the problem (similar to how “ risk ” is often used). Yet, damage is rarely explicitly considered or quantified in the risk analyses (exceptions are: Ubugunov et al. 2011, Hai et al. 2013, Wang et al. 2015). Only one study (Ubugunov et al. 2011) quantified risk by estimating the expected annual damage.
- There is no consensus around the “ hazard ” land degradation. The definitions of land degradation have evolved in historical contexts. This is mirrored in land degradation risk assessment approaches. What is perceived as the threat (i.e., the hazard) ranges from pure soil-related processes to attempts that include a large variety of processes adversely affecting the human-environment system. The fact that the hazard is usually not specified hinders the predictability of hazard occurrence.
- In the majority of studies, there is no clear distinction between processes and drivers, cause and effect, hazard and vulnerability (exceptions are: Ibáñez et al. 2008, Ubugunov et al. 2011, Ali and Abdel Kawy 2013, Hai et al. 2013, Wang et al. 2015, Akbari et al. 2016, Martínez-Valderrama 2020). This hampers the development of quantitative risk projections, as well as a link to decision making and land management. Even in studies that explicitly include hazard and vulnerability terms, a clear differentiation between the two remains difficult (exception is: Wang et al. 2015).
- Temporal dynamics are sometimes considered, but mostly not embedded within a theoretical risk framework. Usually, land degradation is treated as either permanent or a discrete hazard. However, some studies (Ibáñez et al. 2008, Ubugunov et al. 2011, Masoudi and Jokar 2018, Martínez-Valderrama et al. 2020, Masoudi et al. 2021a, 2021b) do include a probabilistic element of risk, facilitating a distinction between present degradation status and future risk. Two of those studies (Ibáñez et al. 2008, Martínez-Valderrama et al. 2020) are based on scenario analyses in state and transition models.
In the following, we propose ideas to approach bridging the gap between land degradation research and DRR concepts in order to stimulate debate and further research on this globally relevant topic. We argue that integrating the already internationally accepted risk framework outlined by the UNDRR could help overcome several of the above-identified shortcomings in land degradation risk assessment approaches and substantially strengthen the link to land management and risk-informed decision making.
First of all, using consistent risk terminology of UNDRR (2022) would promote the transferability of results and the implementation of DRR strategies by decision makers. Second, our literature analysis indicated that it is necessary to clearly differentiate between drivers and processes of land degradation. Only then does a differentiation between hazard and vulnerability, between present degradation status and future risk become possible, thereby promoting a better understanding of risk and an identification of effective entry points for DRR measures (Cardona 2004, Hessel et al. 2014, Masoudi et al. 2021b). Stating the respective perspective on land degradation (e.g., which definition is used) helps to establish clarity about what is actually perceived as a risk. In order to connect to the disaster risk framework of UNDRR, where risk is described as a function of exposure, hazard, and vulnerability, we propose to identify the key land degradation processes that reduce the land’s ecological integrity, productivity, or value to humans as perceived by land users on a local or regional level and treat them as the hazards, henceforth “ degradation hazards ” (Table 2, Fig. 1).
In the next step, the specific drivers of the involved processes as well as the associated ecological and socioeconomic vulnerability (potential damage) should be identified. The adverse effects on the ecosystem (here: “ ecological vulnerability ” ) depend on the bio-physical susceptibility and on the ecological resilience to the degradation hazards. Previous research has demonstrated that human actions (such as land management) have the potential to reduce ecological resilience and that a loss of resilience increases the likelihood of regime shifts toward a less desirable (i.e., degraded) ecosystem state (Holling 1973, Gunderson 2000, Scheffer et al. 2001, Folke et al. 2004, Vetter 2009). Gunderson (2000) argues that if ecosystems shift to a less desirable (i.e., degraded) system state, resource crises occur. Hence, the concept of ecological resilience offers valuable entry points for a quantification of ecological vulnerability to degradation processes and climate variability under different land use options. We propose to build on the concept of ecological resilience to clearly distinguish between rapid climatic drivers (e.g., extreme rainfall events or droughts) of land degradation hazards and gradual drivers of degradation (e.g., land use) that affect the ecological resilience to these degradation hazards and thereby act on the ecological vulnerability (Fig. 2).
Treating the dynamic components of land degradation drivers in two ways, i.e., probabilistically for rapid climatic drivers, and scenario-based for slow anthropogenic drivers and long-term climatic trends, may present a way out of the dilemma of treating land degradation either as a permanent hazard, which yields few options for a probabilistic approach as exemplified in Ubugunov et al. (2011), or a discrete, but unpredictable event in time. Incorporating the steps suggested in Figure 1 into land degradation risk assessment approaches would promote a quantification of the area-specific land degradation risk resulting from concrete degradation hazards in combination with the underlying ecological vulnerability. For a given probability of hazard occurrence, several land management and climate scenarios could then be compared (as shown for flood risk in Thieken et al. 2016). This is an important step toward empirically based projections of land degradation risk and a link to risk-informed decision making in land management. Because the need for a coupling of natural and human vulnerabilities has been stressed in several studies (e.g., Alcántara-Ayala 2002, Turner et al. 2003), a valuable next step would be the inclusion of socioeconomic damage.
CONCLUSIONS
In this paper we aimed at critically analyzing existing land degradation risk assessment approaches and identifying discrepancies with regard to the internationally established risk concept of UNDRR. Key identified shortcomings are that the term “ risk ” is frequently used in its colloquial meaning, and that risk terminology is not consistent across and within studies. A main challenge hindering a link to land management is that there is often no distinction between a degradation status assessment or a degradation monitoring approach and an assessment of future risk. This difficulty is further amplified by a confusion of risk assessment with an assessment of the land’s vulnerability to degradation. Damage is rarely explicitly considered or quantified, and there appears to be no consensus about what the hazard of land degradation contains. In the majority of studies there is no clear distinction between processes and drivers, cause and effect, hazard and vulnerability. Finally, temporal dynamics of land degradation are rarely considered in a manner that promotes a link to land management. However, in more recent studies there appears to be a development toward accounting for the probabilistic nature of degradation risk. This development promotes a distinction between an assessment of present degradation status and future risk. Altogether, this synthesis identified several key limitations of existing land degradation risk assessment approaches, thereby offering valuable entry points for establishing a link to risk-informed land management. Finally, we argue that integrating the already internationally established risk framework outlined by the UNDRR could help overcome several of the above-identified shortcomings. To advance this idea, conceptual ideas toward bridging the gap between land degradation research and DRR strategies were developed. First, we propose to follow consistent risk terminology outlined by UNDRR (UNDRR 2022) when addressing land degradation risk. Second, we emphasize the need to clearly differentiate between hazard, vulnerability, and risk, as well as between drivers and processes of land degradation. By differentiating the various components of risk, existing and very detailed risk assessment frameworks for land degradation such as the frequently applied Environmental Sensitive Areas (ESA) approach could be linked more directly to different stages of disaster risk management (i.e., prevention, preparedness, response, and recovery), as suggested in the Sendai Framework of Disaster Risk Reduction (UNISDR 2015). Further, we propose to clearly distinguish between rapid climatic drivers of land degradation hazards and gradual drivers such as land use affecting the land’s ecological resilience to these hazards and thereby the ecological vulnerability. Hence, effects of different climate and land management scenarios could be compared to assess future land degradation risk. These ideas can be valuable starting points to facilitate further discussions on this globally relevant topic and for the development of effective risk reduction measures for land degradation.
RESPONSES TO THIS ARTICLE
Responses to this article are invited. If accepted for publication, your response will be hyperlinked to the article. To submit a response, follow this link . To read responses already accepted, follow this link .
AUTHOR CONTRIBUTIONS
J.v.K. wrote the article. A.H.T & E.N.P. substantially revised, edited, and critically reviewed all versions of the article. All authors contributed to the objectives, conception, and draft of the article.
ACKNOWLEDGMENTS
This paper is based on a PhD thesis (von Keyserlingk 2021) submitted to TU Berlin, which was conducted within the DFG-Research Training Group NatRiskChange. We thank U. Dolezal for her valuable support in preparing the figures in this article for publication and S. Foerster for fruitful discussions of initial ideas regarding the concept of this article. Finally, we are grateful to the Potsdam Graduate School for their support in proofreading and to the anonymous reviewers for their constructive comments, which considerably helped to strengthen this manuscript.
FUNDING DETAILS This work was supported by the Deutsche Forschungsgemeinschaft (DFG) within the research training group NatRiskChange “ Natural Hazards ad Risks in a Changing World ” [grant numbers GRK 2043/1 and 2043/2].
DATA AVAILABILITY
Data and code sharing is not applicable to this article. The 33 papers analyzed in this synthesis are provided in Table 1 within the paper.
LITERATURE CITED
Abdallah, S., and E. Massoud. 2018. Land degradation risk assessment in Al-Sawda terraces, Kingdom of Saudi Arabia. Arabian Journal of Geosciences 11:599. https://doi.org/10.1007/s12517-018-3956-x
Abu Seif, E., and M. El-Khashab. 2019. Desertification risk assessment of sand dunes in Middle Egypt: a geotechnical environmental study. Arabian Journal for Science and Engineering 44(1):357-375. https://doi.org/10.1007/s13369-018-3343-7
Akbari, M., M. Ownegh, H. R. Asgari, A. Sadoddin, and H. Khosravi. 2016. Desertification risk assessment and management program. Global Journal of Environmental Science and Management 2(4):365-380. https://doi.org/10.22034/gjesm.2016.02.04.006
Alcántara-Ayala, I. 2002. Geomorphology, natural hazards, vulnerability and prevention of natural disasters in developing countries. Geomorphology 47(2-4):107-124. https://doi.org/10.1016/S0169-555X(02)00083-1
Ali, R. R., and W. A. M. Abdel Kawy. 2013. Land degradation risk assessment of El Fayoum depression, Egypt. Arabian Journal of Geosciences 6(8):2767-2776. https://doi.org/10.1007/s12517-012-0524-7
Becerril-Piña, R., C. A. Mastachi-Loza, E. González-Sosa, C. Díaz-Delgado, and K. M. Bâ. 2015. Assessing desertification risk in the semi-arid highlands of central Mexico. Journal of Arid Environments 120:4-13. https://doi.org/10.1016/j.jaridenv.2015.04.006
Cardona, O. D. 2004. The need for rethinking the concepts of vulnerability and risk from a holistic perspective: a necessary review and criticism for effective risk management. Chapter 3 in G. Bankoff, D. Hilhorst, and G. Frerks, editors. Mapping vulnerability: disasters, development and people. Earthscan, Routledge, London, UK.
Dregne, H. E. 2002. Land degradation in the drylands. Arid Land Research and Management 16(2):99-132. https://doi.org/10.1080/153249802317304422
Felgentreff, C., and T. Glade. 2007. Naturrisiken und Sozialkatastrophen. Spektrum Akademischer Verlag, Heidelberg, Germany
Folke, C., S. Carpenter, B. Walker, M. Scheffer, T. Elmqvist, L. Gunderson, and C. S. Holling. 2004. Regime shifts, resilience, and biodiversity in ecosystem management. Annual Review of Ecology, Evolution, and Systematics 35(1):557-581. https://doi.org/10.1146/annurev.ecolsys.35.021103.105711
Garg, P. K., and A. R. Harrison. 1992. Land degradation and erosion risk analysis in S.E. Spain: a geographic information system approach. Catena 19(5):411-425. https://doi.org/10.1016/0341-8162(92)90041-9
Gunderson, L. H. 2000. Ecological resilience - in theory and application. Annual Review of Ecology and Systematics 31(1):425-439. https://doi.org/10.1146/annurev.ecolsys.31.1.425
Hai, L. T., A. Gobin, and L. Hens. 2013. Risk assessment of desertification for Binh Thuan Province, Vietnam. Human and Ecological Risk Assessment: An International Journal 19(6):1544-1556. https://doi.org/10.1080/10807039.2012.716688
Hastings, A., and D. B. Wysham. 2010. Regime shifts in ecological systems can occur with no warning. Ecology Letters 13(4):464-472. https://doi.org/10.1111/j.1461-0248.2010.01439.x
Hessel, R., M. S. Reed, N. Geeson, C. J. Ritsema, G. van Lynden, C. A. Karavitis, G. Schwilch, V. Jetten, P. Burger, M. J. van der Werff ten Bosch, S. Verzandvoort, E. van den Elsen, and K. Witsenburg. 2014. From framework to action: the DESIRE approach to combat desertification. Environmental Management 54(5):935-950. https://doi.org/10.1007/s00267-014-0346-3
Holling, C. S. 1973. Resilience and stability of ecological systems. Annual Review of Ecology and Systematics 4(1):1-23. https://doi.org/10.1146/annurev.es.04.110173.000245
Holling, C. S. 1996. Engineering resilience versus ecological resilience. Pages 31-43 in P. C. Schulze, editor. Engineering within ecological constraints. National Academies, Washington, D.C., USA.
Hsu, E. L. 2019. Must disasters be rapidly occurring? The case for an expanded temporal typology of disasters. Time & Society 28(3):904-921. https://doi.org/10.1177/0961463X17701956
Ibáñez, J., J. Martínez-Valderrama, and J. Puigdefábregas. 2008. Assessing desertification risk using system stability condition analysis. Ecological Modelling 213(2):180-190. https://doi.org/10.1016/j.ecolmodel.2007.11.017
Intergovernmental Panel on Climate Change (IPCC). 2012. Managing the risks of extreme events and disasters to advance climate change adaptation: special report of the Intergovernmental Panel on Climate Change. C. B. Field, V. Barros, T. F. Stocker, and Q. Dahe, editors. Cambridge University Press, Cambridge, UK. https://doi.org/10.1017/CBO9781139177245
Intergovernmental Science-Policy Platform on Biodiversity and Ecosystem Services (IPBES). 2018. The IPBES assessment report on land degradation and restoration. L. Montanarella, R. Scholes, and A. Brainich, editors. Secretariat of the IPBES, Bonn, Germany. https://doi.org/10.5281/zenodo.3237392
Kaplan, S., and B. J. Garrick. 1981. On the quantitative definition of risk. Risk Analysis 1(1):11-27. https://doi.org/10.1111/j.1539-6924.1981.tb01350.x
Karamesouti, M., P. Panagos, and C. Kosmas. 2018. Model-based spatio-temporal analysis of land desertification risk in Greece. Catena 167:266-275. https://doi.org/10.1016/j.catena.2018.04.042
Karavitis, C., D. Tsesmelis, P. Oikonomou, O. Kairis, C. Kosmas, V. Fassouli, C. Ritsema, R. Hessel, V. Jetten, N. Moustakas, B. Todorovic, N. Skondras, C. Vasilakou, S. Alexandris, E. Kolokytha, D. Stamatakos, R. Stricevic, E. Chatzigeorgiadis, J. Brandt, N. Geeson, and G. Quaranta. 2020. A desertification risk assessment decision support tool (DRAST). Catena 187:104413. https://doi.org/10.1016/j.catena.2019.104413
Karssenberg, D., M. F. P. Bierkens, and M. Rietkerk. 2017. Catastrophic shifts in semiarid vegetation-soil systems may unfold rapidly or slowly. American Naturalist 190(6). https://doi.org/10.1086/694413
Kéfi, S., V. Guttal, W. A. Brock, S. R. Carpenter, A. M. Ellison, V. N. Livina, D. A. Seekell, M. Scheffer, E. H. van Nes, and V. Dakos. 2014. Early warning signals of ecological transitions: methods for spatial patterns. PLoS ONE 9(3):e92097. https://doi.org/10.1371/journal.pone.0092097
Land Degradation Assessment in Drylands (LADA). 2011. Land degradation assessment in drylands: methodology and results. Food and Agriculture Organization of the United Nations, Rome, Italy. https://www.fao.org/3/i3241e/i3241e.pdf
Ladisa, G., M. Todorovic, and G. Trisorio Liuzzi. 2012. A GIS-based approach for desertification risk assessment in Apulia region, SE Italy. Physics and Chemistry of the Earth, Parts A/B/C 49:103-113. https://doi.org/10.1016/j.pce.2011.05.007
Leopold, L. B., F. E. Clarke, B. B. Hanshaw, and J. R. Balsley. 1971. A procedure for evaluating environmental impact. U.S. Geological Survey, Washington, D.C., USA. https://doi.org/10.3133/cir645
Mäler, K.-G. 2000. Development, ecological resources and their management: a study of complex dynamic systems. European Economic Review 44:645-665. https://doi.org/10.1016/S0014-2921(00)00043-X
Martínez-Valderrama, J., J. Ibáñez, F. Alcalá, and S. Martínez. 2020. SAT: a software for assessing the risk of desertification in Spain. Scientific Programming 2020:7563928. https://doi.org/10.1155/2020/7563928
Masoudi, M., M. Elhaeesahar, and A. Cerdà . 2021a. Risk assessment of land degradation (RALDE) in Khuzestan Province, Iran. Eurasian Soil Science 54(8):1228-1240. https://doi.org/10.1134/S1064229321080135
Masoudi, M., and P. Jokar. 2018. Risk assessment of desertification using GIS in upper and lower reaches of Mond basin, Iran. AUC Geographica 53(2):156-166. https://doi.org/10.14712/23361980.2018.16
Masoudi, M., M. Vahedi, and A. Cerda. 2021b. Risk assessment of land degradation (RALDE) model. Land Degradation & Development 32(9):2861-2874. https://doi.org/10.1002/ldr.3883
Matthewman, S. 2015. Disasters, risks and revelation: making sense of our times. Palgrave Macmillan, London, UK. https://doi.org/10.1057/9781137294265
Merz, B., F. Elmer, and A. H. Thieken. 2009. Significance of "high probability/low damage" versus "low probability/high damage" flood events. Natural Hazards and Earth System Sciences 9(3):1033-1046. https://doi.org/10.5194/nhess-9-1033-2009
Millennium Ecosystem Assessment (MA). 2005. Ecosystems and human well-being: synthesis. Island Press, Washington, D.C., USA.
Nachtergaele, F., R. Biancalani, S. Bunning, and H. George. 2010. Land degradation assessment: the LADA approach. Pages 72-75 in 19th World Congress of Soil Science, Symposium 3.1.1, Further development of soil evaluation methods, Soil Solutions for a Changing World, 1–6 August, Brisbane, Australia.
Nkonya, E., A. Mirzabaev, and J. von Braun, editors. 2016. Economics of land degradation and improvement - a global assessment for sustainable development. Springer, Cham, Switzerland. https://doi.org/10.1007/978-3-319-19168-3
Olsson, L., H. Barbosa, S. Bhadwal, A. Cowie, K. Delusca, D. Flores-Renteria, K. Hermans, E. Jobbagy, W. Kurz, D. Li, D. J. Sonwa, L. Stringer. 2019. Land degradation. Chapter 4 in P. R. Shukla, J. Skea, E. Calvo Buendia, V. Masson-Delmotte, H.-O. Pörtner, D. C. Roberts, P. Zhai, R. Slade, S. Connors, R. van Diemen, M. Ferrat, E. Haughey, S. Luz, S. Neogi, M. Pathak, J. Petzold, J. Portugal Pereira, P. Vyas, E. Huntley, K. Kissick, M. Belkacemi, J. Malley, editors. Climate change and land: an IPCC special report on climate change, desertification, land degradation, sustainable land management, food security, and greenhouse gas fluxes in terrestrial ecosystems. https://www.ipcc.ch/srccl/chapter/chapter-4/
Pishyar, S., H. Khosravi, A. Tavili, A. Malekian, and S. Sabourirad. 2020. A combined AHP- and TOPSIS-based approach in the assessment of desertification disaster risk. Environmental Modeling & Assessment 25(2):219-229. https://doi.org/10.1007/s10666-019-09676-8
Plate, E. J., and B. Merz, editors. 2001. Naturkatastrophen: Ursachen, Auswirkungen, Vorsorge. E. Schweizerbart’sche Verlagsbuchhandlung (Nägele u. Obermüller), Stuttgart, Germany.
Popkin, G. 2014. On the edge. Science 345(6204):1552-1554. https://doi.org/10.1126/science.345.6204.1552
Rietkerk, M., and J. van de Koppel. 1997. Alternate stable states and threshold effects in semi-arid grazing systems. Oikos 79(1):69-76. https://doi.org/10.2307/3546091
Roques, K. G., T. G. O'Connor, and A. R. Watkinson. 2001. Dynamics of shrub encroachment in an African savanna: relative influences of fire, herbivory, rainfall and density dependence. Journal of Applied Ecology 38(2):268-280. https://doi.org/10.1046/j.1365-2664.2001.00567.x
Rubio, J. L., and E. Bochet. 1998. Desertification indicators as diagnosis criteria for desertification risk assessment in Europe. Journal of Arid Environments 39(2):113-120. https://doi.org/10.1006/jare.1998.0402
Salunkhe, S., A. Bera, S. Rao, V. Venkataraman, U. Raj, and Y. Murthy. 2018. Evaluation of indicators for desertification risk assessment in part of Thar Desert Region of Rajasthan using geospatial techniques. Journal of Earth System Science 127:116. https://doi.org/10.1007/s12040-018-1016-2
Salvati, L., C. Kosmas, O. Kairis, C. Karavitis, S. Acikalin, A. Belgacem, A. Solé-Benet, M. Chaker, V. Fassouli, C. Gokceoglu, H. Gungor, R. Hessel, H. Khatteli, A. Kounalaki, A. Laouina, F. Ocakoglu, M. Ouessar, C. Ritsema, M. Sghaier, H. Sonmez, H. Taamallah, L. Tezcan, and J. de Vente. 2015. Unveiling soil degradation and desertification risk in the Mediterranean basin: a data mining analysis of the relationships between biophysical and socioeconomic factors in agro-forest landscapes. Journal of Environmental Planning and Management 58(10):1789-1803. https://doi.org/10.1080/09640568.2014.958609
Salvati, L., and M. Zitti. 2009. Convergence or divergence in desertification risk? Scale-based assessment and policy implications in a Mediterranean country. Journal of Environmental Planning and Management 52(7):957-971. https://doi.org/10.1080/09640560903181220
Salvati, L., M. Zitti, and T. Ceccarelli. 2008. Integrating economic and environmental indicators in the assessment of desertification risk: a case study. Applied Ecology and Environmental Research 6(1):129-138. https://doi.org/10.15666/aeer/0601_129138
Santini, M., G. Caccamo, A. Laurenti, S. Noce, and R. Valentini. 2010. A multi-component GIS framework for desertification risk assessment by an integrated index. Applied Geography 30(3):394-415. https://doi.org/10.1016/j.apgeog.2009.11.003
Scheffer, M. 1990. Multiplicity of stable states in freshwater systems. Hydrobiologia 200:475-486. https://doi.org/10.1007/BF02530365
Scheffer, M., S. Carpenter, J. A. Foley, C. Folke, and B. Walker. 2001. Catastrophic shifts in ecosystems. Nature 413(6856):591-596. https://doi.org/10.1038/35098000
Scheffer, M., and S. R. Carpenter. 2003. Catastrophic regime shifts in ecosystems: linking theory to observation. Trends in Ecology & Evolution 18(12):648-656. https://doi.org/10.1016/j.tree.2003.09.002
Scheffer, M., S. R. Carpenter, V. Dakos, and E. H. van Nes. 2015. Generic indicators of ecological resilience: Inferring the chance of a critical transition. Annual Review of Ecology, Evolution, and Systematics 46(1):145-167. https://doi.org/10.1146/annurev-ecolsys-112414-054242
Staupe-Delgado, R. 2019. Progress, traditions and future directions in research on disasters involving slow-onset hazards. Disaster Prevention and Management: An International Journal 28(5):623-635. https://doi.org/10.1108/DPM-11-2018-0358
Taguas, E. V., E. Carpintero, and J. L. Ayuso. 2013. Assessing land degradation risk through the long-term analysis of erosivity: a case study in southern Spain. Land Degradation & Development 24(2):179-187. https://doi.org/10.1002/ldr.1119
Thieken, A. H., H. Cammerer, C. Dobler, J. Lammel, and F. Schöberl. 2016. Estimating changes in flood risks and benefits of non-structural adaptation strategies - a case study from Tyrol, Austria. Mitigation and Adaptation Strategies for Global Change 21(3):343-376. https://doi.org/10.1007/s11027-014-9602-3
Tomaselli, R. 1977. The degradation of the Mediterranean maquis. Ambio 6(6):356-362. https://www.jstor.org/stable/4312322
Tombolini, I., A. Colantoni, G. Renzi, A. Sateriano, A. Sabbi, N. Morrow, and L. Salvati. 2016. Lost in convergence, found in vulnerability: a spatially-dynamic model for desertification risk assessment in Mediterranean agro-forest districts. Science of The Total Environment 569-570:973-981. https://doi.org/10.1016/j.scitotenv.2016.06.049
Türkes, M., T. Öztaş, E. Tercan, G. Erpul, A. Karagöz, O. Dengiz, O. Doğan, K. Şahin, and B. Avcioğlu. 2020. Desertification vulnerability and risk assessment for Turkey via an analytical hierarchy process model. Land Degradation & Development 31(2):205-214. https://doi.org/10.1002/ldr.3441
Turnbull, L., J. Wainwright, and R. E. Brazier. 2008. A conceptual framework for understanding semi-arid land degradation: ecohydrological interactions across multiple-space and time scales. Ecohydrology 1(1):23-34. https://doi.org/10.1002/eco.4
Turner II, B. L., R. E. Kasperson, P. A. Matson, J. J. McCarthy, R. W. Corell, L. Christensen, N. Eckley, J. X. Kasperson, A. Luers, M. L. Martello, et al. 2003. A framework for vulnerability analysis in sustainability science. Proceedings of the National Academy of Sciences 100(14):8074-8079. https://doi.org/10.1073/pnas.1231335100
Ubugunov, L. L., A. I. Kulikov, and M. A. Kulikov. 2011. On the application of risk analysis technology for assessment of the ecological hazard of desertification (by the example of Republic of Buryatia). Contemporary Problems of Ecology 4(2):178-185. https://doi.org/10.1134/S1995425511020093
United Nations Convention to Combat Desertification (UNCCD). 1994. United Nations Convention to Combat Desertification (UNCCD) in those countries experiencing serious drought and/or desertification, particularly in Africa. United Nations General Assembly, Paris, France. https://www.unccd.int/sites/default/files/2022-02/English_0.pdf
United Nations Convention to Combat Desertification (UNCCD). 2020. United Nations Convention to Combat Desertification. UNCCD, Bonn, Germany. https://www.unccd.int
United Nations International Strategy for Disaster Risk Reduction (UNISDR). 2015. Sendai framework for disaster risk reduction 2015-2030. The Framework was adopted at the Third UN World Conference on Disaster Risk Reduction in Sendai, Japan, on March 18, 2015. https://www.undrr.org/publication/sendai-framework-disaster-risk-reduction-2015-2030
United Nations Office for Disaster Risk Reduction (UNDRR). 2019. Global assessment report on disaster risk reduction. UNDRR, Geneva, Switzerland.
United Nations Office for Disaster Risk Reduction (UNDRR). 2022. Terminology on disaster risk reduction. UNDRR, Geneva, Switzerland. https://www.undrr.org/terminology
Uzuner, C., and O. Dengiz. 2020. Desertification risk assessment in Turkey based on environmentally sensitive areas. Ecological Indicators 114:106295. https://doi.org/10.1016/j.ecolind.2020.106295
Vetter, S. 2009. Drought, change and resilience in South Africa's arid and semi-arid rangelands. South African Journal of Science 105:29. https://doi.org/10.4102/sajs.v105i1/2.35
Vieira, R., J. Tomasella, A. Barbosa, M. Martins, D. Rodriguez, F. Rezende, F. Carriello, and M. Santana. 2021. Desertification risk assessment in Northeast Brazil: current trends and future scenarios. Land Degradation & Development 32(1):224-240. https://doi.org/10.1002/ldr.3681
von Keyserlingk, J. 2021. Ecological resilience and the risk of land degradation in a dry Mediterranean rangeland. Dissertation. Technische Universität Berlin, Berlin, Germany. https://doi.org/10.14279/depositonce-12496
von Keyserlingk, J., M. de Hoop, A. G. Mayor, S. C. Dekker, M. Rietkerk, and S. Foerster. 2021. Resilience of vegetation to drought: studying the effect of grazing in a Mediterranean rangeland using satellite time series. Remote Sensing of Environment 255:112270. https://doi.org/10.1016/j.rse.2020.112270
Vorovencii, I. 2015. Assessing and monitoring the risk of desertification in Dobrogea, Romania, using Landsat data and decision tree classifier. Environmental Monitoring and Assessment 187:204. https://doi.org/10.1007/s10661-015-4428-3
Vorovencii, I. 2016. Assessing and monitoring the risk of land degradation in Baragan Plain, Romania, using spectral mixture analysis and Landsat imagery. Environmental Monitoring and Assessment 188:439. https://doi.org/10.1007/s10661-016-5446-5
Vorovencii, I. 2017. Applying the change vector analysis technique to assess the desertification risk in the south-west of Romania in the period 1984–2011. Environmental Monitoring and Assessment 189:524. https://doi.org/10.1007/s10661-017-6234-6
Wang, Y., J. Zhang, E. Guo, and Z. Sun. 2015. Fuzzy comprehensive evaluation-based disaster risk assessment of desertification in Horqin Sand Land, China. International Journal of Environmental Research and Public Health 12(2):1703-1725. https://doi.org/10.3390/ijerph120201703
Weinzierl, T., J. Wehberg, J. Böhner, and O. Conrad. 2016. Spatial assessment of land degradation risk for the Okavango River catchment, Southern Africa. Land Degradation & Development 27(2):281-294. https://doi.org/10.1002/ldr.2426
Zaidi, R. Z. 2018. Beyond the Sendai indicators: application of a cascading risk lens for the improvement of loss data indicators for slow-onset hazards and small-scale disasters. International Journal of Disaster Risk Reduction 30:306-314. https://doi.org/10.1016/j.ijdrr.2018.03.022
Figures, Tables, & Appendices ×

Fig. 1. Suggested steps for a land degradation risk analysis at ecosystem level, integrating the risk framework of the United Nations Office for Disaster Risk Reduction and the concept of ecological resilience.

Fig. 2. Conceptualization of the proposed risk-resilience model for land degradation. Based on a combination of the “ball-in-a-cup” model for ecological resilience (adapted from Popkin 2014) and the classical risk function developed within disaster risk research. Figure adapted from von Keyserlingk (2021).

Table 1. United Nations Office for Disaster Risk Reduction terminology related to disaster risk reduction (UNDRR 2022).
Table 2. proposed identification of risk components and indicators for land degradation risk at ecosystem level, integrating the disaster risk framework of the united nations office for disaster risk reduction. table adapted from von keyserlingk (2021)..
Click on a keyword to view more articles on that topic.
Submit a response to this article
- Frontiers in Forests and Global Change
- Forest Management
- Research Topics
Land Degradation and Forest Management
Total Downloads
Total Views and Downloads
About this Research Topic
Land degradation, meaning any reduction or loss in the biological or economic productive capacity of the land resource base, is a global problem. Land, water, and forest resources can become degraded due to poor and often short-sighted management. In this context, land degradation neutrality (LDN) is an ...
Keywords : Land degradation neutrality, Landuse dynamics, Forest resilience, Climate change and invasion, Forest and livelihood
Important Note : All contributions to this Research Topic must be within the scope of the section and journal to which they are submitted, as defined in their mission statements. Frontiers reserves the right to guide an out-of-scope manuscript to a more suitable section or journal at any stage of peer review.
Topic Editors
Topic coordinators, recent articles, submission deadlines, participating journals.
Manuscripts can be submitted to this Research Topic via the following journals:
total views
- Demographics
No records found
total views article views downloads topic views
Top countries
Top referring sites, about frontiers research topics.
With their unique mixes of varied contributions from Original Research to Review Articles, Research Topics unify the most influential researchers, the latest key findings and historical advances in a hot research area! Find out more on how to host your own Frontiers Research Topic or contribute to one as an author.
What is desertification and why is it important to understand?
Currently, around 2 billion people live in drylands, which are most prone to desertification, according to Earth.org. Image: REUTERS/Ivan Alvarado
.chakra .wef-1c7l3mo{-webkit-transition:all 0.15s ease-out;transition:all 0.15s ease-out;cursor:pointer;-webkit-text-decoration:none;text-decoration:none;outline:none;color:inherit;}.chakra .wef-1c7l3mo:hover,.chakra .wef-1c7l3mo[data-hover]{-webkit-text-decoration:underline;text-decoration:underline;}.chakra .wef-1c7l3mo:focus,.chakra .wef-1c7l3mo[data-focus]{box-shadow:0 0 0 3px rgba(168,203,251,0.5);} Andrea Willige
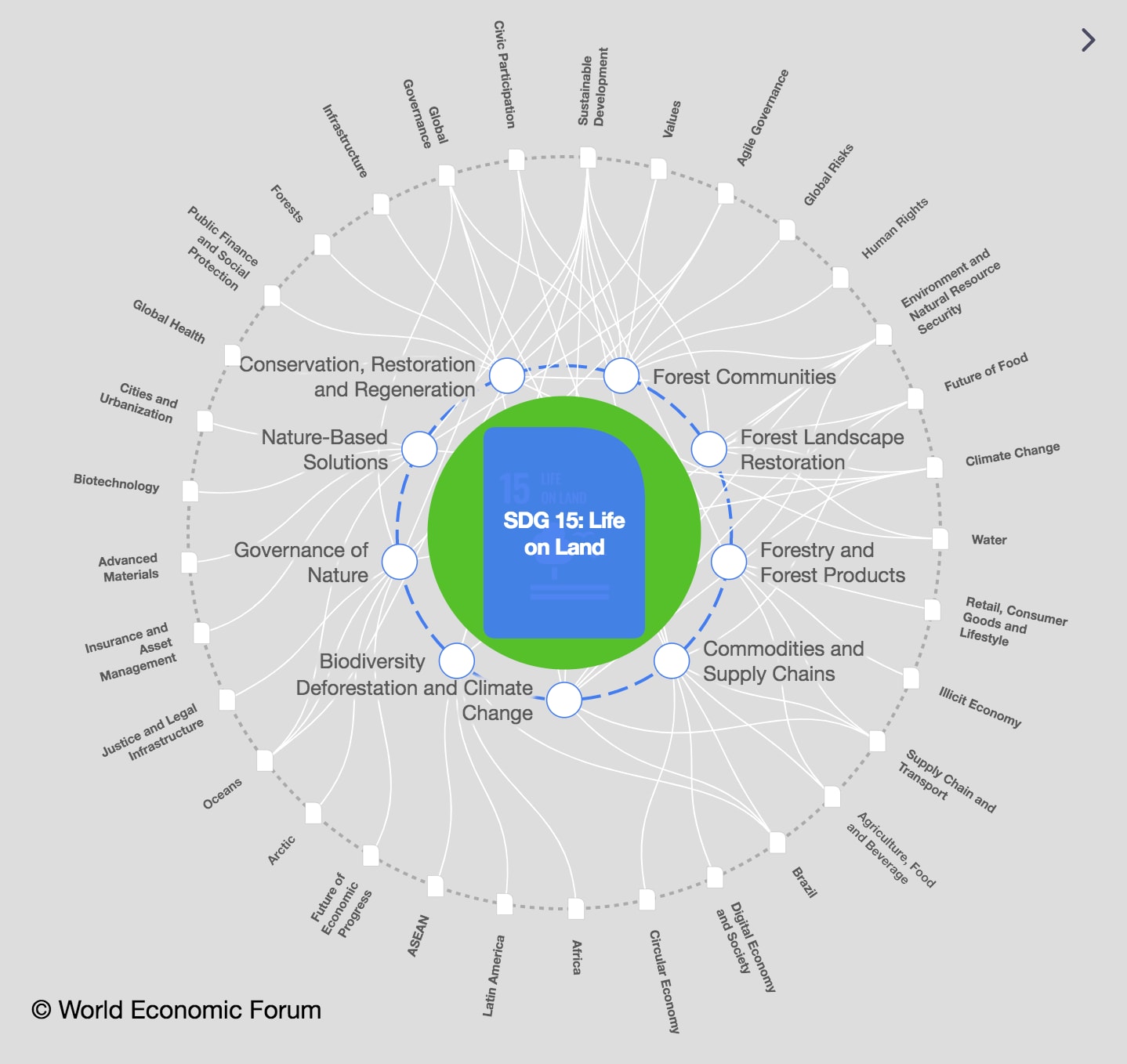
.chakra .wef-9dduvl{margin-top:16px;margin-bottom:16px;line-height:1.388;font-size:1.25rem;}@media screen and (min-width:56.5rem){.chakra .wef-9dduvl{font-size:1.125rem;}} Explore and monitor how .chakra .wef-15eoq1r{margin-top:16px;margin-bottom:16px;line-height:1.388;font-size:1.25rem;color:#F7DB5E;}@media screen and (min-width:56.5rem){.chakra .wef-15eoq1r{font-size:1.125rem;}} SDG 15: Life on Land is affecting economies, industries and global issues
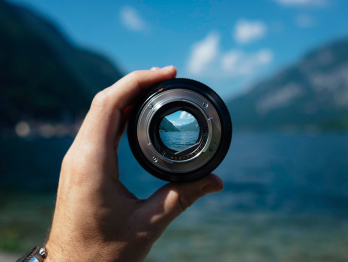
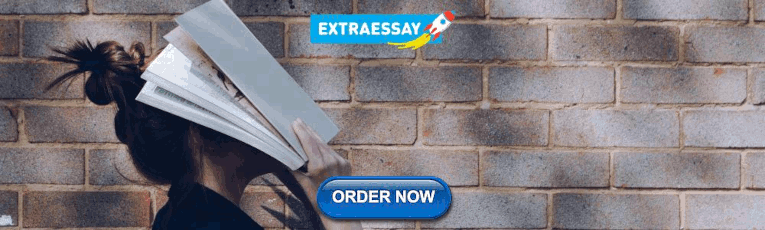
.chakra .wef-1nk5u5d{margin-top:16px;margin-bottom:16px;line-height:1.388;color:#2846F8;font-size:1.25rem;}@media screen and (min-width:56.5rem){.chakra .wef-1nk5u5d{font-size:1.125rem;}} Get involved with our crowdsourced digital platform to deliver impact at scale
Stay up to date:, sdg 15: life on land.
- Factors including climate change, deforestation, overgrazing and unsustainable agricultural practices are increasingly turning our world’s drylands into deserts.
- Degradation of productive soil along with the loss of biodiversity, bodies of water and vegetation also impacts human life, leading to poverty, food and water scarcity and poor health.
- But 2024 could become a seminal year for the fight against desertification, with a series of events including the World Economic Forum’s Special Meeting and COP16 focused on tackling the issue.
When we think of deserts, regions such as the Middle East, Northern Africa or Central Asia may spring to mind. But growing desertification in the wake of climate change is increasingly drawing ever wider circles across the globe, increasing steadily . United Nations’ latest data, as presented by 126 Parties in their 2022 national reports, show that 15.5% of land is now degraded, an increase of 4% in as many years.
But this could become a seminal year for the fight against desertification, with two major events scheduled for 2024 in Saudi Arabia to mobilize support. Tackling this growing problem will be a major focus at the World Economic Forum’s forthcoming Special Meeting on Global Collaboration, Growth and Energy for Development in May, and the16th session of the Conference of the Parties to the UN Convention to Combat Desertification (UNCCD) COP16 in December.
The UNCCD is one of the three Rio Conventions, along with the UN Framework Convention on Climate Change and the Convention on Biological Diversity.
What does desertification mean for our planet and people, and how can we mitigate against it?
Have you read?
These start-ups are helping to make life in the sahel more sustainable, why investing in land is a business imperative for a sustainable future, women's land rights: a key to combating desertification and drought, what is desertification and what causes it.
Desertification is a type of land degradation in which an already relatively dry land area becomes increasingly arid , degrading productive soil and losing its bodies of water, biodiversity and vegetation cover.
It is driven by a combination of factors, including climate change, deforestation, overgrazing and unsustainable agricultural practices .
The issue reaches far beyond deserts like the Sahara, Kalahari or Gobi deserts. The UNCCD says that 100 million hectares of productive land are degraded each year . Droughts are becoming more common, and three-quarters of people are expected to face water scarcity by 2050.
Currently, around 2 billion people live in drylands, which are most prone to desertification , according to Earth.org.
Among the most affected regions are Africa and Eastern and Central Asia.
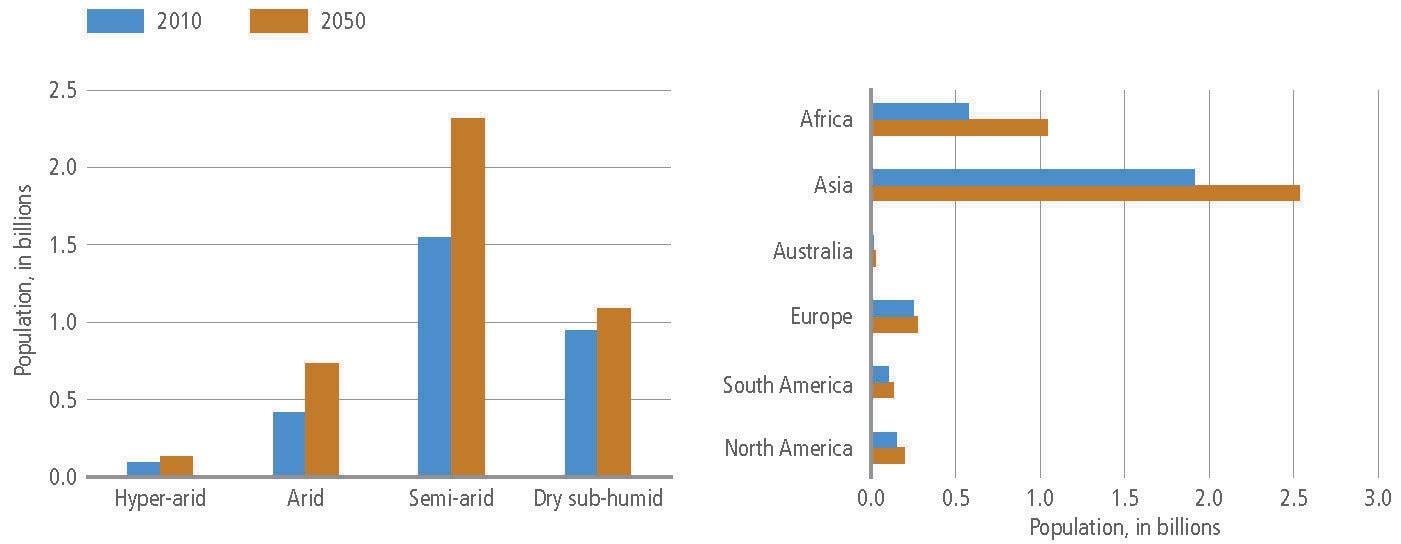
Who is most affected by desertification?
In Africa, some 40 million people are living in severe drought conditions already , according to the World Economic Forum report Quantifying the Impact of Climate Change on Human Health 2024.
And, in Asia, China, Uzbekistan, and Kyrgyzstan are among the countries that have seen temperatures soaring, according to Earth.org. While some of these areas had been classed as having a desert climate since the 1980s, desertification has continued, leading to hotter, wetter climates. In the mountains, a lack of snow has led to the gradual disappearance of glaciers, threatening water security that affects both people and agriculture.
Biodiversity loss and climate change are occurring at unprecedented rates, threatening humanity’s very survival. Nature is in crisis, but there is hope. Investing in nature can not only increase our resilience to socioeconomic and environmental shocks, but it can help societies thrive.
There is strong recognition within the Forum that the future must be net-zero and nature-positive. The Nature Action Agenda initiative, within the Centre for Nature and Climate , is an inclusive, multistakeholder movement catalysing economic action to halt biodiversity loss by 2030.
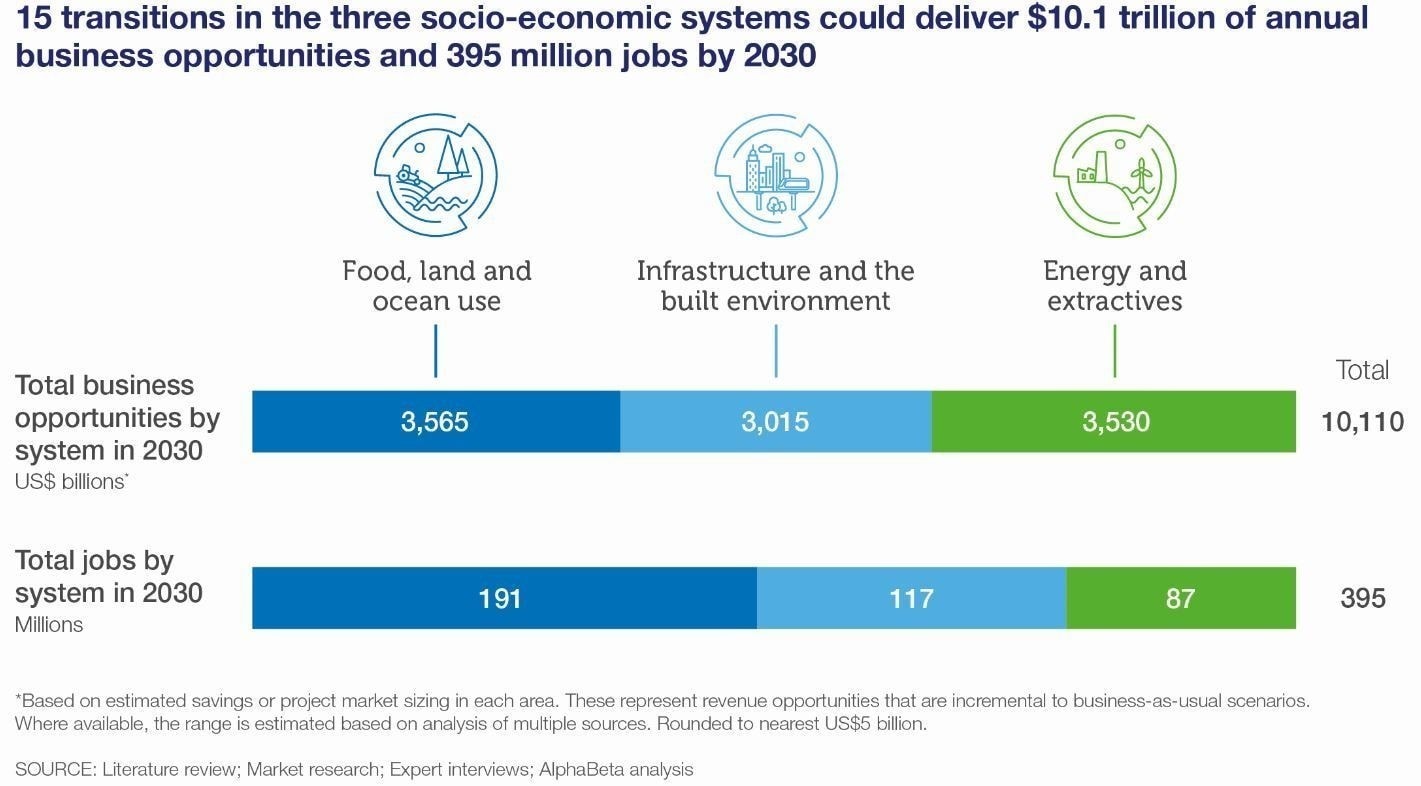
The Nature Action Agenda is enabling business and policy action by:
Building a knowledge base to make a compelling economic and business case for safeguarding nature, showcasing solutions and bolstering research through the publication of the New Nature Economy Reports and impactful communications.
Catalysing leadership for nature-positive transitions through multi-stakeholder communities such as Champions for Nature that takes a leading role in shaping the net-zero, nature-positive agenda on the global stage.
Scaling up solutions in priority socio-economic systems through BiodiverCities by 2030 , turning cities into engines of nature-positive development; Financing for Nature , unlocking financial resources through innovative mechanisms such as high-integrity Biodiversity Credits Market ; and Sector Transitions to Nature Positive , accelerating sector-specific priority actions to reduce impacts and unlock opportunities.
Supporting an enabling environment by ensuring implementation of the Kunming-Montreal Global Biodiversity Framework and mobilizing business voices calling for ambitious policy actions in collaboration with Business for Nature .
But land degradation also affects more temperate regions. In the US, nearly 40% of the lower 48 states are facing drought, the Forum’s report says, quoting statistics from the US National Integrated Drought Information System.
Southern Europe has seen some of its worst droughts in recent years. In Spain, desertification and overexploitation have severely affected what’s known as “Europe’s kitchen garden”. The European Union has flagged the vulnerability of its southern members to desertification in recent years, pointing not only to Spain but also Portugal, Italy, Greece, Cyprus, Bulgaria and Romania.
What are the impacts of desertification?
According to the UNCCD, around 500 million people live in desertified areas .
They can experience exacerbated poverty, lack of food security and poor health due to malnutrition and lack of access to clean water. They are also more vulnerable to climate change and extreme weather such as droughts and natural disasters. With their livelihoods at stake and a greater risk of conflict over declining resources , they may also face forced migration.
One of the most prominent examples of desertification is the Aralkum desert in central Asia. In the 1960s, the area was covered by the fourth-largest lake in the world, the Aral Sea. Since then, it has shrunk to a tenth of its former size, with only three small, highly salty lakes remaining. In Soviet times, its waters were used for irrigating a semi-desert region to grow cotton, leading to a drop in water levels. Climate change added further impetus to this over time, turning the dry seabed into a salt-covered desert, leaving fishing boats stranded, rusting and livelihoods destroyed.
How can we mitigate against desertification?
There are a wide variety of approaches to address desertification , with many programmes underway around the globe.
Reforestation and afforestation can help revive degraded soil. In Uzbekistan, a regreening programme has planted trees and shrubs across one million hectares along the Aral desert. This includes the black saxual shrub, which is highly drought resistant and can fix salt and sand, stopping it from being swept up and carried inland by sandstorms.
In the Sahel and Sahara region in Africa, the “Great Green Wall” – launched in 2007 by the African Union – aims to restore plant life across 100 million hectares of degraded land. Involving 22 African countries, this initiative will revive the land, store more than 220 million tonnes of carbon and create 10 million jobs by 2030.
Another big part of tackling land degradation is introducing sustainable land management practices, ranging from agroforestry to sustainable grazing and can also improve crop yields and livelihoods.
Water management practices such as rainwater harvesting, drip-water irrigation and planting drought-resistant crops can address the impact of water scarcity.
Other remedial steps include re-vegetation and restoring natural habitats such as wetlands or entire river beds.
The World Economic Forum’s Special Meeting in Saudi Arabia will see a series of announcements and sessions on the topic of desertification, including support for Saudi Arabia as the host of COP16 and joint work between the Forum and Saudi Arabia to compile a programme for December’s event.
Don't miss any update on this topic
Create a free account and access your personalized content collection with our latest publications and analyses.
License and Republishing
World Economic Forum articles may be republished in accordance with the Creative Commons Attribution-NonCommercial-NoDerivatives 4.0 International Public License, and in accordance with our Terms of Use.
The views expressed in this article are those of the author alone and not the World Economic Forum.
Related topics:
The agenda .chakra .wef-n7bacu{margin-top:16px;margin-bottom:16px;line-height:1.388;font-weight:400;} weekly.
A weekly update of the most important issues driving the global agenda
.chakra .wef-1dtnjt5{display:-webkit-box;display:-webkit-flex;display:-ms-flexbox;display:flex;-webkit-align-items:center;-webkit-box-align:center;-ms-flex-align:center;align-items:center;-webkit-flex-wrap:wrap;-ms-flex-wrap:wrap;flex-wrap:wrap;} More on Climate Action .chakra .wef-17xejub{-webkit-flex:1;-ms-flex:1;flex:1;justify-self:stretch;-webkit-align-self:stretch;-ms-flex-item-align:stretch;align-self:stretch;} .chakra .wef-nr1rr4{display:-webkit-inline-box;display:-webkit-inline-flex;display:-ms-inline-flexbox;display:inline-flex;white-space:normal;vertical-align:middle;text-transform:uppercase;font-size:0.75rem;border-radius:0.25rem;font-weight:700;-webkit-align-items:center;-webkit-box-align:center;-ms-flex-align:center;align-items:center;line-height:1.2;-webkit-letter-spacing:1.25px;-moz-letter-spacing:1.25px;-ms-letter-spacing:1.25px;letter-spacing:1.25px;background:none;padding:0px;color:#B3B3B3;-webkit-box-decoration-break:clone;box-decoration-break:clone;-webkit-box-decoration-break:clone;}@media screen and (min-width:37.5rem){.chakra .wef-nr1rr4{font-size:0.875rem;}}@media screen and (min-width:56.5rem){.chakra .wef-nr1rr4{font-size:1rem;}} See all
How Indigenous expertise is empowering climate action: A case study from Oceania
Amanda Young and Ginelle Greene-Dewasmes
April 23, 2024
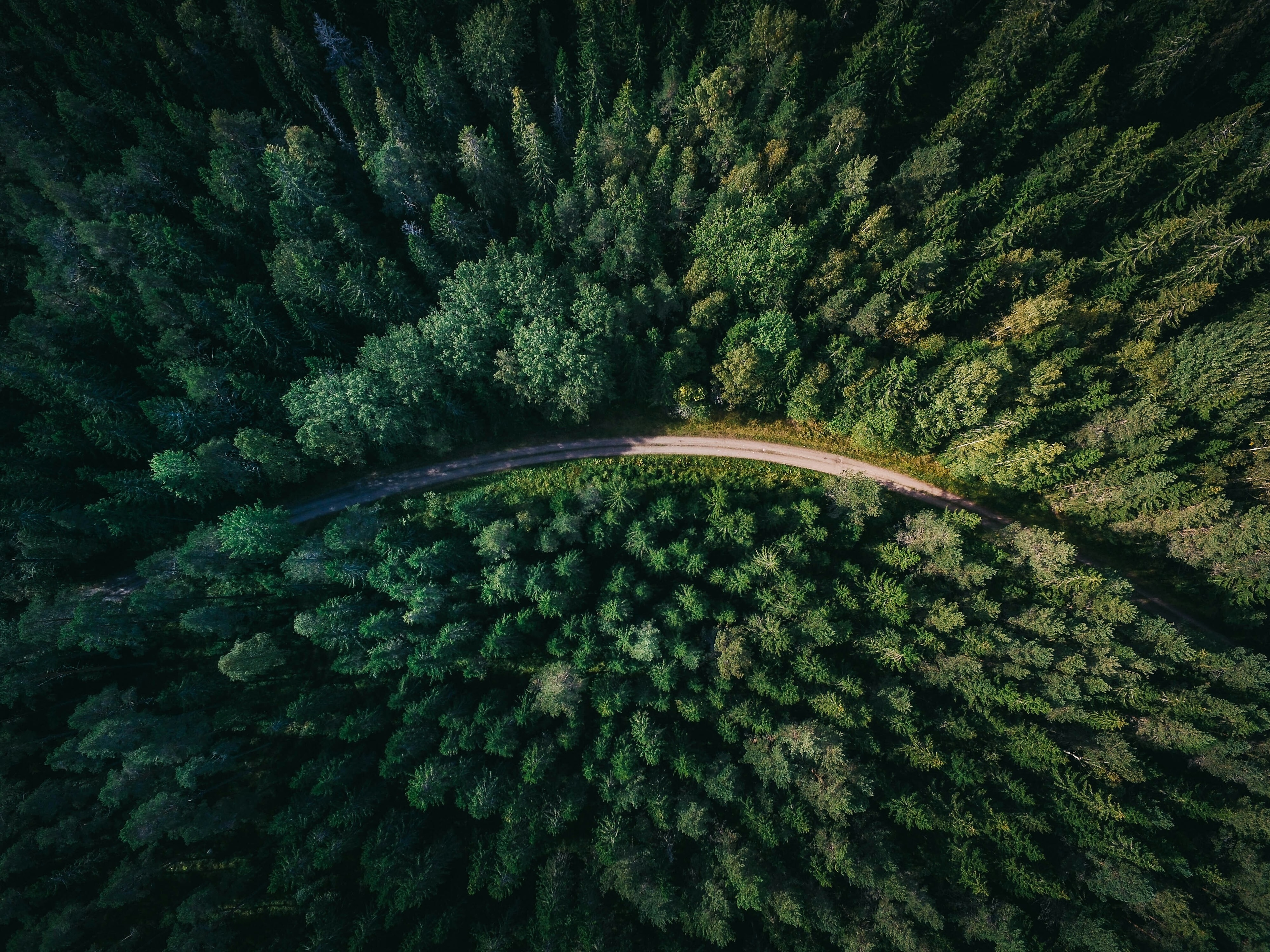
Global forest restoration goals can be achieved with youth-led ecopreneurship
Agustin Rosello, Anali Bustos, Fernando Morales de Rueda, Jennifer Hong and Paula Sarigumba
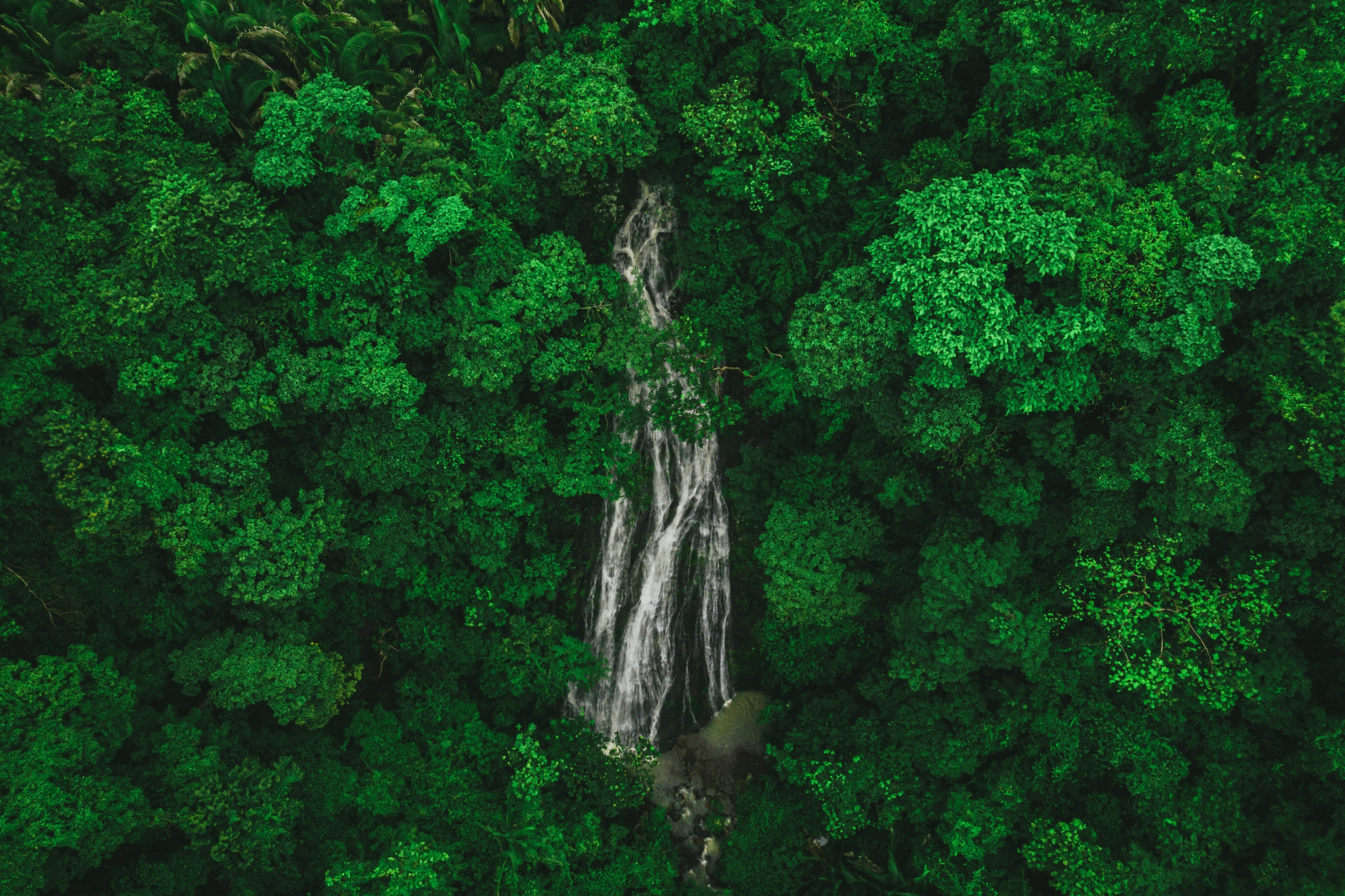
The planet’s outlook is in our hands. Which future will we incentivize?
Carlos Correa
April 22, 2024
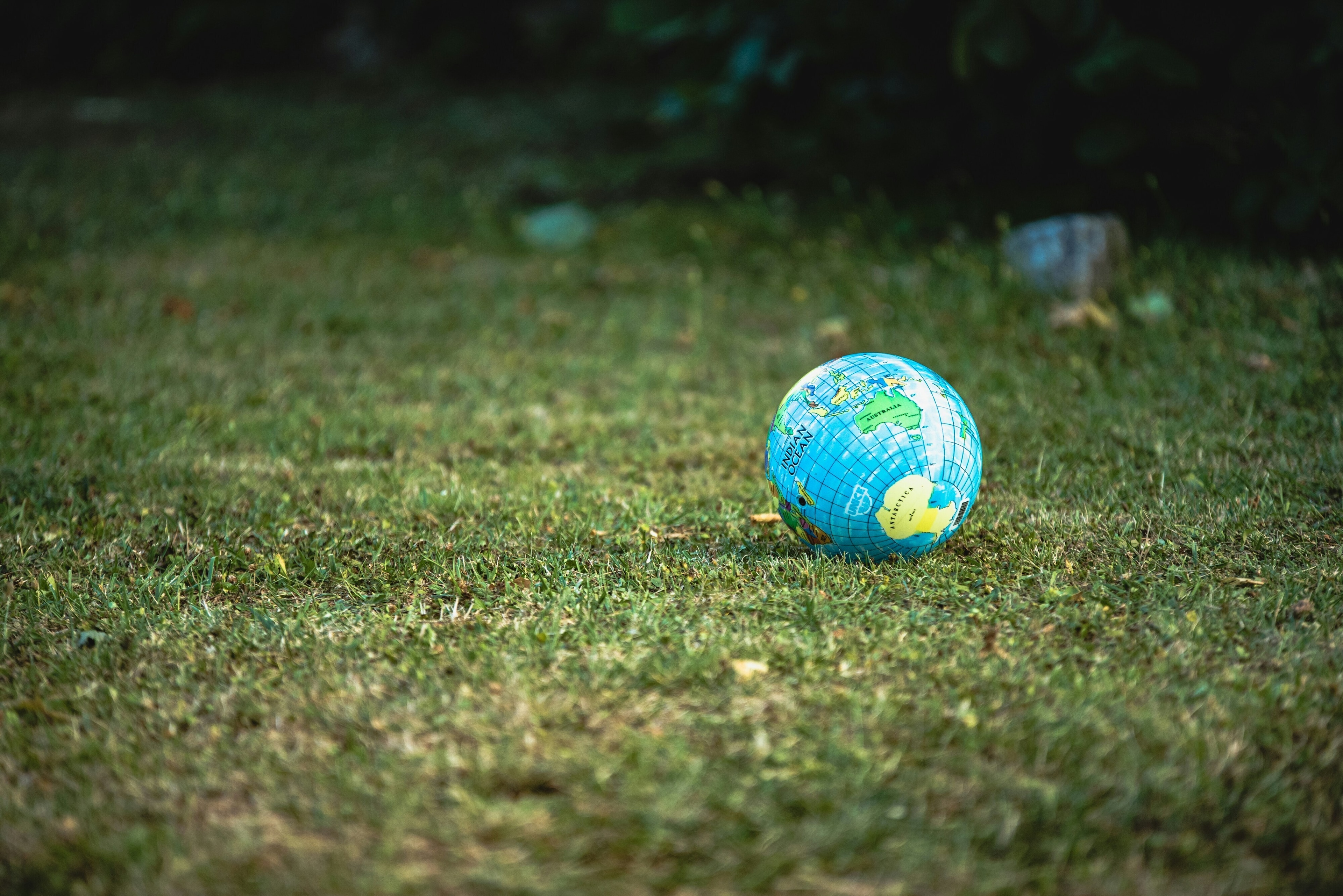
This Earth Day we consider the impact of climate change on human health
Shyam Bishen and Annika Green
Earth Day: We are almost certainly all eating plastics, says report, and other nature and climate stories you need to read this week
Johnny Wood
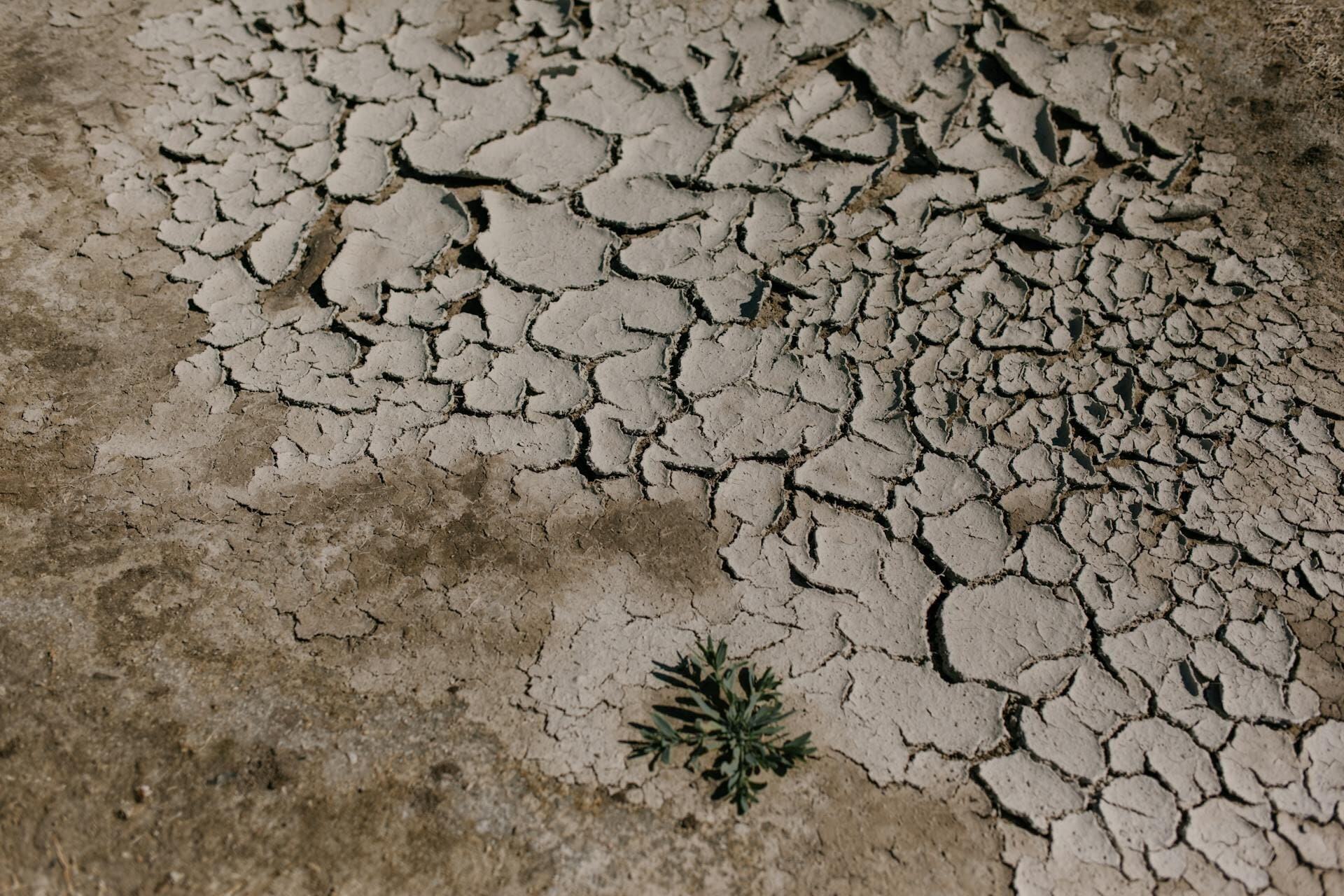
$400 billion debt burden: Emerging economies face climate action crisis
Libby George
April 19, 2024
Spatial Variations of the Activity of 137 Cs and the Contents of Heavy Metals and Petroleum Products in the Polluted Soils of the City of Elektrostal
- DEGRADATION, REHABILITATION, AND CONSERVATION OF SOILS
- Open access
- Published: 15 June 2022
- Volume 55 , pages 840–848, ( 2022 )
Cite this article
You have full access to this open access article
- D. N. Lipatov 1 ,
- V. A. Varachenkov 1 ,
- D. V. Manakhov 1 ,
- M. M. Karpukhin 1 &
- S. V. Mamikhin 1
1460 Accesses
2 Citations
Explore all metrics
The levels of specific activity of 137 Cs and the contents of mobile forms (1 M ammonium acetate extraction) of heavy metals (Zn, Cu, Ni, Co, Cr, Pb) and petroleum products were studied in the upper soil horizon of urban landscapes of the city of Elektrostal under conditions of local radioactive and chemical contamination were studied. In the soils within a short radius (0–100 m) around the heavy engineering plant, the specific activity of 137 Cs and the contents of mobile forms of Pb, Cu, and Zn were increased. The lognormal distribution law of 137 Cs was found in the upper (0–10 cm) soil layer; five years after the radiation accident, the specific activity of 137 Cs varied from 6 to 4238 Bq/kg. The coefficients of variation increased with an increase in the degree of soil contamination in the following sequence: Co < Ni < petroleum products < Cr < 137 Cs < Zn < Pb < Cu ranging from 50 to 435%. Statistically significant direct correlation was found between the specific activity of 137 Cs and the contents of mobile forms of Pb, Cu, and Zn in the upper horizon of urban soils, and this fact indicated the spatial conjugacy of local spots of radioactive and polymetallic contamination in the studied area. It was shown that the specific activity of 137 Cs, as well as the content of heavy metals and petroleum products in the upper layer (0–10 cm) of the soils disturbed in the course of decontamination, earthwork and reclamation is reduced.
Similar content being viewed by others
Accumulation and migration of heavy metals in soils of the rostov region, south of russia.
Olga S. Bezuglova, Sergey N. Gorbov, … Marina N. Dubinina
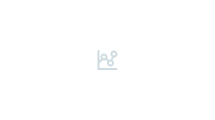
Geographical Features of Pollution of the Territory of Yakutia With Cesium-137
P. I. Sobakin, A. P. Chevychelov & Ya. R. Gerasimov
Activity Concentration of Natural Radionuclides and Total Heavy Metals Content in Soils of Urban Agglomeration
Avoid common mistakes on your manuscript.
INTRODUCTION
Contaminants migrate and accumulate in urban ecosystems under the impact of both natural and technogenic factors. The processes of technogenic migration of 137 Cs are most pronounced in radioactively contaminated territories. It was found in urboecological studies that the intensity of sedimentation of aerosol particles containing radionuclides and heavy metals is determined by the types of the surfaces of roofs, walls, roads, lawns, and parks and by their position within the urban wind field [ 12 , 26 ]. Traffic in the cities results in significant transport of dust and associated contaminants and radionuclides [ 15 , 24 ]. During decontamination measures in the areas of Chernobyl radioactive trace, not only the decrease in the level of contamination but also the possibility of secondary radioactive contamination because of the transportation of contaminated soil particles by wind or water, or anthropogenic transfer of transferring of ground were observed [ 5 , 6 ]. Rainstorm runoff and hydrological transport of dissolved and colloidal forms of 137 Cs can result in the accumulation of this radionuclide in meso- and microdepressions, where sedimentation takes place [ 10 , 16 ]. Different spatial distribution patterns of 137 Cs in soils of particular urban landscapes were found in the city of Ozersk near the nuclear fuel cycle works [ 17 ]. Natural character of 137 Cs migration in soils of Moscow forest-parks and a decrease in its specific activity in industrial areas have been revealed [ 10 ]. Determination of the mean level and parameters of spatial variations of 137 Cs in soils is one of primary tasks of radioecological monitoring of cities, including both unpolluted (background) and contaminated territories.
Emissions and discharges from numerous sources of contamination can cause the accumulation of a wide range of toxicants in urban soils: heavy metals (HMs), oil products (OPs), polycyclic aromatic hydrocarbons (PAHs), and other chemical substances. Soil contamination by several groups of toxicants is often observed in urban landscapes [ 20 , 23 ] because of the common contamination source or close pathways of the migration of different contaminants. A comprehensive analysis of contamination of urban soils by radionuclides and heavy metals has been performed in some studies [ 21 , 25 ]. The determination of possible spatial interrelationships between radioactive and chemical contaminations in urban soils is an important problem in urban ecology.
A radiation accident took place in the Elektrostal heavy engineering works (EHEW) in April 2013: a capacious source of 137 Cs entered the smelt furnace, and emission of radioactive aerosols from the aerating duct into the urban environment took place. The activity of molten source was estimated at about 1000–7000 Ci [ 14 ]. The area of contamination in the territory of the plant reached 7500 m 2 . However, radioactive aerosols affected a much larger area around the EHEW, including Krasnaya and Pervomaiskaya streets, and reached Lenin Prospect.
Geochemical evaluation of contamination of the upper soil horizon in the city of Elektrostal was carried out in 1989–1991. This survey indicated the anomalies of concentrations of wolfram, nickel, molybdenum, chromium, and other heavy metals related to accumulation of alloying constituent and impurities of non-ferrous metals in the emissions of steelmaking works [ 19 ].
The aim of our work was to determine the levels of specific activity of 137 Cs, concentrations of mobile forms of heavy metals (Zn, Cu, Ni, Co, Cr, and Pb) and oil products in the upper soil horizons in different urban landscapes of the city of Elektrostal under the conditions of local radioactive and chemical contamination.
Author information
Authors and affiliations.
Lomonosov Moscow State University, 119991, Moscow, Russia
D. N. Lipatov, V. A. Varachenkov, D. V. Manakhov, M. M. Karpukhin & S. V. Mamikhin
You can also search for this author in PubMed Google Scholar
Corresponding author
Correspondence to D. N. Lipatov .
Ethics declarations
The authors declare that they have no conflicts of interest.
Additional information
Translated by T. Chicheva
Rights and permissions
Open Access. This article is licensed under a Creative Commons Attribution 4.0 International License, which permits use, sharing, adaptation, distribution and reproduction in any medium or format, as long as you give appropriate credit to the original author(s) and the source, provide a link to the Creative Commons license, and indicate if changes were made. The images or other third party material in this article are included in the article’s Creative Commons license, unless indicated otherwise in a credit line to the material. If material is not included in the article’s Creative Commons license and your intended use is not permitted by statutory regulation or exceeds the permitted use, you will need to obtain permission directly from the copyright holder. To view a copy of this license, visit http://creativecommons.org/licenses/by/4.0/ .
Reprints and permissions
About this article
Lipatov, D.N., Varachenkov, V.A., Manakhov, D.V. et al. Spatial Variations of the Activity of 137 Cs and the Contents of Heavy Metals and Petroleum Products in the Polluted Soils of the City of Elektrostal. Eurasian Soil Sc. 55 , 840–848 (2022). https://doi.org/10.1134/S1064229322060072
Download citation
Received : 21 October 2021
Revised : 22 December 2021
Accepted : 30 December 2021
Published : 15 June 2022
Issue Date : June 2022
DOI : https://doi.org/10.1134/S1064229322060072
Share this article
Anyone you share the following link with will be able to read this content:
Sorry, a shareable link is not currently available for this article.
Provided by the Springer Nature SharedIt content-sharing initiative
- urban soils
- urban ecosystems
- radiation monitoring
- decontamination
- Urban Technosols
- Find a journal
- Publish with us
- Track your research

An official website of the United States government
Here’s how you know
Official websites use .gov A .gov website belongs to an official government organization in the United States.
Secure .gov websites use HTTPS A lock ( Lock A locked padlock ) or https:// means you’ve safely connected to the .gov website. Share sensitive information only on official, secure websites.

Groundwater sustainability and land subsidence in California’s Central Valley
- More information: Publisher Index Page (via DOI)
- Open Access Version: Publisher Index Page
- Download citation as: RIS | Dublin Core
More From Forbes
Protecting our health starts with our planet: a heart surgeon’s view.
- Share to Facebook
- Share to Twitter
- Share to Linkedin
As a heart surgeon, I made major decisions daily guided by what medical science told me. And, right now, scientific evidence increasingly shows that nature – the land, water, plants, and animals around us – is fundamental to our personal health.
But we should be concerned. Because scientific evidence also indicates that with society’s progressive economic growth (a positive thing), our planet’s biodiversity is declining . We are losing green spaces, freshwater sources, and plant and animal species at an alarming rate. And climate change further accelerates this. Indeed, if we don’t act now to better prioritize conservation, soon there might not be much nature as we know it left. This is not to be alarmist; it is just where we are heading. Our personal health — that of you and me and generations to follow— and the health of our communities, will irreversibly suffer. And our overall quality of life will diminish alongside it.
Right now, scientific evidence increasingly shows that nature – the land, water, plants, and animals ... [+] around us – is fundamental to our personal health.
To more clearly and more rationally discuss climate change and biodiversity loss, and to bring significantly more people to the table to engage in the conversation of solutions and actions, I strongly believe we should more aggressively frame the discussion in terms of its impact on our physical and mental health. People care about their health, and the health of their children and grandchildren, and if they understand how climate change, land degradation, and species loss is detrimental to their health, they will engage, they will respond, and they will act.
So let’s view climate change and the environment through the lens of individual and public health. We are already starting to see the negative impact of a continually warming planet on our health. This isn’t surprising. Think about the growing number of extreme heat waves, catastrophic weather events, and days with poor air quality that we have witnessed every year over the past decade. Each of these directly and indirectly influences our ability to live a healthy and fulfilling life and for our communities to thrive.
And this is exactly why I, a former heart and lung surgeon, am serving as the chair of the global board of The Nature Conservancy , the world’s largest nonprofit dedicated to protecting nature and tackling climate change with solutions that are good for our planet and good for people. It’s not just conservation for conservation's sake; it’s for people, to make their lives better. I spent 20 years studying health science and personally delivering health services, so I understand peoples’ health. I see how nature and climate are inextricably linked to personal health.
Sixers Justified In Fury Toward Officiating After Game 2 Meltdown Vs. Knicks
The trump media stock price djt is about to adjust down by 22 7, sh gun episode 10 review a powerful finale but not what i was expecting.
Why is conservation important to your health?
On average our mental, physical, and emotional health in the U.S. ranks behind other developed countries. Take life expectancy, for example. The U.S. is not in the top 10 or even the top 20 when it comes to the average number of years we can expect to live. In fact, we rank 26 th with a life expectancy of 77.5 years . The most advanced and wealthiest nation in the world ranks 26 th in the health of its people! And compared to similarly sized, wealthy countries, we have the absolute lowest life expectancy despite outspending every one of them on healthcare.
In terms of our mental health specifically, we are also far behind. The United Nations 2024 annual World Happiness Report placed the U.S. 23 rd in the world in happiness, falling from 15 th place in last year’s rankings. Indeed, our country has the highest suicide rate of any wealthy nation (double that of the United Kingdom).
For a country that spends nearly 20% of our GDP on healthcare, these numbers are especially shocking. And it’s not just because of a lack of doctors in the U.S., or uneven access to healthcare, or inadequate health insurance that our health outcomes rank so poorly. It’s an issue that runs much deeper than that and stems from how we interact with and behave in the world around us. And our environment plays a fundamental role.
Prioritizing conservation – better understanding and more actively protecting nature and the natural ... [+] world we live in – is a great way for each of us to start changing this narrative and improving our health and wellbeing.
That is why prioritizing conservation – better understanding and more actively protecting nature and the natural world we live in – is a great way for each of us to start changing this narrative and improving our health and wellbeing. It’s not just better for the planet, it’s better for us as individuals, and our families and communities.
A Case Study: Heart Health and Nature
Here is where it is personal for me. One clinical area where the intersection of our environment and our health can be clearly seen is my own medical specialty of heart and lung disease. Cardiovascular disease is consistently one of the leading causes of death worldwide, accounting for 17.9 million deaths each year. It is the leading cause of death for women and men here at home. This acute and chronic condition is greatly impacted by the health of our environment, especially when it comes to heat events and air pollution.
Heat : Heat events are the top annual weather-related killer in the U.S. And rising temperatures are making extreme heat waves more frequent and more intense. As our average temperatures continue to trend upward - this past winter was the warmest on record - so does the already high risk of heat-related adverse health effects for those with cardiovascular disease. The human body simply was not made to thrive in extremely high and rapidly elevating temperatures . And our bodies’ evolution can’t keep up with the rapid pace of temperature escalation. Because of this, heat waves result in a range of devastating physical consequences like heat stroke, heart attacks, cardiovascular and respiratory stress, dehydration, and stroke.
And research also shows that rising temperatures greatly affect our mental and emotional health as well. Hotter days result in an uptick in reported anxiety, anger, suicide attempts, and overdose deaths . A 2019 study by the National Bureau of Economic Research found a 5.7% increase in violent crime on days above 85 degrees Fahrenheit than on cooler days in Los Angeles.
Air Pollution : Air pollution is the fourth leading cause of disease and death worldwide. Research shows that exposure to poor air quality due to particle pollution increases the risk of heart-related events. In fact, a recent Global Burden of Disease (GBD) study estimates that air pollution resulted in 9 million deaths globally , 61.9% of which was due to cardiovascular disease. Similarly in 2022, researchers published findings suggesting a connection between air pollution and lung cancer , and air pollution has also recently been associated with dementia .
Events such as wildfires directly affect air quality. Climate change has doubled the number the number of large fires over the last three decades. As fire seasons grow longer and more severe due to drier conditions, chronic illness and the significant costs related to smoke exposure are surging (premature deaths and hospital admissions accounted for more than half of the total cost of the fires).
Lung Health : Our lung function is also greatly impacted by our environment. Hotter and drier conditions lead to a greater risk of wildfires which release microscopic particles in smoke and make it difficult to breathe (especially for those with preexisting conditions like asthma, or heart and lung disease). And in areas where climate change has increased rainfall and floods, moisture is leading to heightened risk of mold which is also bad for our lungs. Further, higher temperatures cause plants to produce more pollen and allergens and to do so over a greater range. As a result, allergy seasons, and their related morbidity to individuals, are lasting even longer and geographically expanding to new areas.
The science is clear: the natural world around is inextricably linked to our health – the health of our bodies, the health of our communities, and the health of our planet. And when nature suffers, so, too, does our personal health. But the good news is that, by this same token when nature thrives, so do we. That’s right. Mother Nature is medicinal. I saw it in my medical practice and the scientific literature supports it . And protecting and conserving nature can be a great way to not only protect our health but improve it. Economic growth and progress can and will continue to flourish, but as responsible citizens we should insist that they do so with careful attention to the balanced, long-term sustainability of nature and biodiversity. And I should add, with my former political hat on, this is not a Democrat or Republican issue, it is a public health and wellbeing issue for us all.
One of the great benefits of being outside in nature is exercise – which is good for our hearts, lungs, and mental wellbeing. Heart disease is linked to a lack of physical activity. Because of this, spending time out in nature can be a successful prevention strategy for cardiac-related events like heart attacks, high blood pressure, and strokes. When it comes to our mental and emotional health, just being in nature has been shown to have measurable, positive effects. Studies show that cortisol —our stress hormone — levels are significantly reduced with something as simple as a 20-minute walk in a local park.
Paying attention to nature, harnessing the tremendous power of biodiversity, and conserving our lands and waters can greatly improve our health. It’s good for our planet. And it’s good for people. Each of us has an important role to play in preserving nature and biodiversity. Our health – and that of generations to come – depends on answering this call.
- Editorial Standards
- Reprints & Permissions

Current time by city
For example, New York
Current time by country
For example, Japan
Time difference
For example, London
For example, Dubai
Coordinates
For example, Hong Kong
For example, Delhi
For example, Sydney
Geographic coordinates of Elektrostal, Moscow Oblast, Russia
City coordinates
Coordinates of Elektrostal in decimal degrees
Coordinates of elektrostal in degrees and decimal minutes, utm coordinates of elektrostal, geographic coordinate systems.
WGS 84 coordinate reference system is the latest revision of the World Geodetic System, which is used in mapping and navigation, including GPS satellite navigation system (the Global Positioning System).
Geographic coordinates (latitude and longitude) define a position on the Earth’s surface. Coordinates are angular units. The canonical form of latitude and longitude representation uses degrees (°), minutes (′), and seconds (″). GPS systems widely use coordinates in degrees and decimal minutes, or in decimal degrees.
Latitude varies from −90° to 90°. The latitude of the Equator is 0°; the latitude of the South Pole is −90°; the latitude of the North Pole is 90°. Positive latitude values correspond to the geographic locations north of the Equator (abbrev. N). Negative latitude values correspond to the geographic locations south of the Equator (abbrev. S).
Longitude is counted from the prime meridian ( IERS Reference Meridian for WGS 84) and varies from −180° to 180°. Positive longitude values correspond to the geographic locations east of the prime meridian (abbrev. E). Negative longitude values correspond to the geographic locations west of the prime meridian (abbrev. W).
UTM or Universal Transverse Mercator coordinate system divides the Earth’s surface into 60 longitudinal zones. The coordinates of a location within each zone are defined as a planar coordinate pair related to the intersection of the equator and the zone’s central meridian, and measured in meters.
Elevation above sea level is a measure of a geographic location’s height. We are using the global digital elevation model GTOPO30 .
Elektrostal , Moscow Oblast, Russia

- Investigation
- Contact us
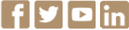

For the first time Rosatom Fuel Division supplied fresh nuclear fuel to the world’s only floating nuclear cogeneration plant in the Arctic
The fuel was supplied to the northernmost town of Russia along the Northern Sea Route.
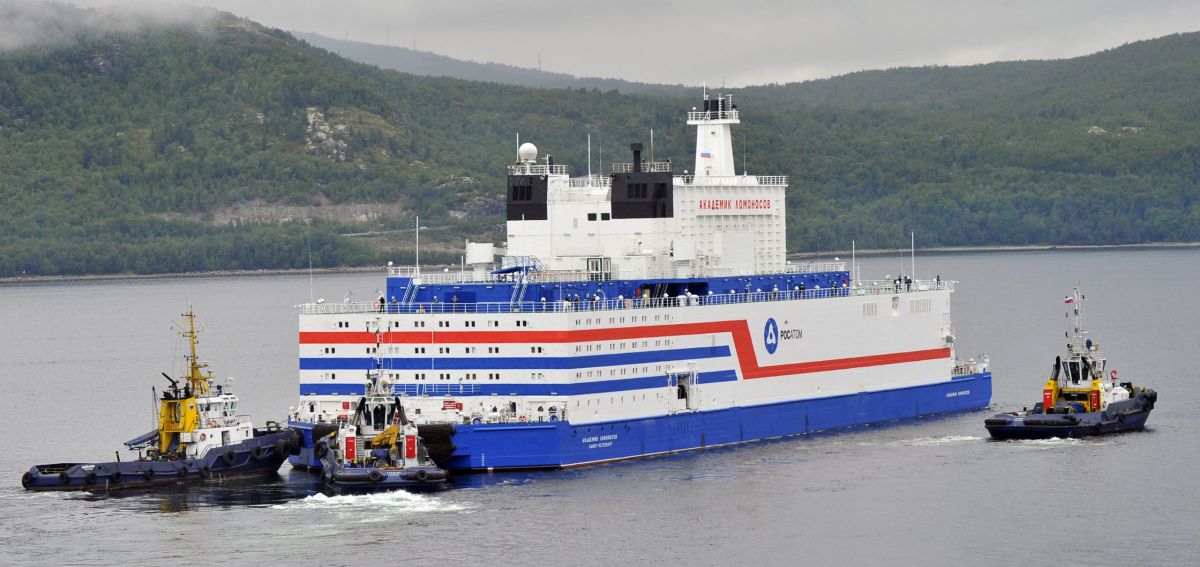
The first in the history of the power plant refueling, that is, the replacement of spent nuclear fuel with fresh one, is planned to begin before 2024. The manufacturer of nuclear fuel for all Russian nuclear icebreakers, as well as the Akademik Lomonosov FNPP, is Machinery Manufacturing Plant, Joint-Stock Company (MSZ JSC), a company of Rosatom Fuel Company TVEL that is based in Elektrostal, Moscow Region.
The FNPP includes two KLT-40S reactors of the icebreaking type. Unlike convenient ground-based large reactors (that require partial replacement of fuel rods once every 12-18 months), in the case of these reactors, the refueling takes place once every few years and includes unloading of the entire reactor core and loading of fresh fuel into the reactor.
The cores of KLT-40 reactors of the Akademik Lomonosov floating power unit have a number of advantages compared to the reference ones: a cassette core was used for the first time in the history of the unit, which made it possible to increase the fuel energy resource to 3-3.5 years between refuelings, and also reduce the fuel component of the electricity cost by one and a half times. The FNPP operating experience formed the basis for the designs of reactors for nuclear icebreakers of the newest series 22220. Three such icebreakers have been launched by now.
For the first time the power units of the Akademik Lomonosov floating nuclear power plant were connected to the grid in December 2019, and put into commercial operation in May 2020. The supply of nuclear fuel from Elektrostal to Pevek and its loading into the second reactor is planned for 2024. The total power of the Akademik Lomonosov FNPP, supplied to the coastal grid of Pevek without thermal energy consumption on shore, is about 76 MW, being about 44 MW in the maximum thermal power supply mode. The FNPP generated 194 million kWh according to the results of 2023. The population of Pevek is just a little more than 4 thousand, while the FNPP has a potential for supplying electricity to a city with a population of up to 100 thousand people. After the FNPP commissioning two goals were achieved. These include first of all the replacement of the retiring capacities of the Bilibino NPP, which has been operating since 1974, as well as the Chaunskaya TPP, which has already been operating for more than 70 years. Secondly, energy is supplied to the main mining companies in western Chukotka in the Chaun-Bilibino energy hub a large ore and metal cluster, including gold mining companies and projects related to the development of the Baimsk ore zone. In September 2023, a 110 kilovolt power transmission line with a length of 490 kilometers was put into operation, connecting the towns of Pevek and Bilibino. The line increased the reliability of energy supply from the FNPP to both Bilibino consumers and mining companies, the largest of which is the Baimsky GOK. The comprehensive development of the Russian Arctic is a national strategic priority. To increase the NSR traffic is of paramount importance for accomplishment of the tasks set in the field of cargo shipping. This logistics corridor is being developed due regular freight voyages, construction of new nuclear-powered icebreakers and modernization of the relevant infrastructure. Rosatom companies are actively involved in this work. Rosatom Fuel Company TVEL (Rosatom Fuel Division) includes companies fabricating nuclear fuel, converting and enriching uranium, manufacturing gas centrifuges, conducting researches and producing designs. As the only nuclear fuel supplier to Russian NPPs, TVEL supplies fuel for a total of 75 power reactors in 15 countries, for research reactors in nine countries, as well as for propulsion reactors of the Russian nuclear fleet. Every sixth power reactor in the world runs on TVEL fuel. Rosatom Fuel Division is the world’s largest producer of enriched uranium and the leader on the global stable isotope market. The Fuel Division is actively developing new businesses in chemistry, metallurgy, energy storage technologies, 3D printing, digital products, and decommissioning of nuclear facilities. TVEL also includes Rosatom integrators for additive technologies and electricity storage systems. Rosenergoatom, Joint-Stock Company is part of Rosatom Electric Power Division and one of the largest companies in the industry acting as an operator of nuclear power plants. It includes, as its branches, 11 operating NPPs, including the FNPP, the Scientific and Technical Center for Emergency Operations at NPPs, Design and Engineering as well as Technological companies. In total, 37 power units with a total installed capacity of over 29.5 GW are in operation at 11 nuclear power plants in Russia. Machinery Manufacturing Plant, Joint-Stock Company (MSZ JSC, Elektrostal) is one of the world’s largest manufacturers of fuel for nuclear power plants. The company produces fuel assemblies for VVER-440, VVER-1000, RBMK-1000, BN-600,800, VK-50, EGP-6; powders and fuel pellets intended for supply to foreign customers. It also produces nuclear fuel for research reactors. The plant belongs to the TVEL Fuel Company of Rosatom.

Rosatom obtained a license for the first land-based SMR in Russia
On April 21, Rosenergoatom obtained a license issued by Rostekhnadzor to construct the Yakutsk land-based SMR in the Ust-Yansky District of the Republic of Sakha (Yakutia).

ROSATOM and FEDC agree to cooperate in the construction of Russia's first onshore SNPP
ROSATOM and FEDC have signed a cooperation agreement to build Russia's first onshore SNPP in Yakutia.
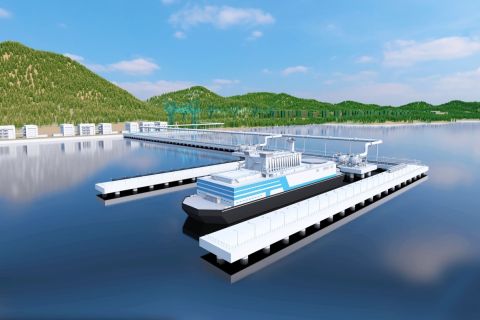
Rosatom develops nuclear fuel for modernized floating power units
Rosatom has completed the development of nuclear fuel for the RITM-200S small modular reactor designed for the upgraded floating power units.
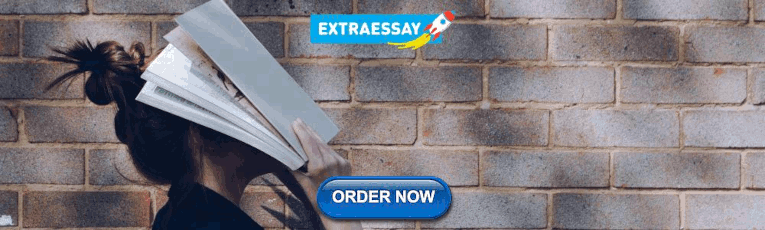
IMAGES
VIDEO
COMMENTS
UN Environment-led land degradation projects across 162 countries, including $55 million in projects currently under implementation. The UN Environment-GEF land degradation portfolio currently comprises 54 projects, ranging from smaller enabling activities to medium- and full-size projects. Many of these are in Africa, acknowledging the severe ...
demands, are the key drivers of land degradation. o There is a need for urgent action on land degradation now given future projections of population growth and climate change impacts (as the scenario work done for the UNCCD GLO demonstrated). o It is essential to avoid, reduce and reverse land degradation (LDN) to overcome these challenges.
Abstract. Land degradation has been a subject of academic research all across the world and still an important global. issue in the twenty- rst century. Land is an essential non-renewable resource ...
Degraded Land - resulting in reduced productivity ~ 5 billion ha (@ 43% of Earth's vegetated surface) ~ @ 1/3 due to overgrazing ~ 3.6 billion ha associated with desertification ~ a major cause is overgrazing ~ 0.5 billion ha due to tree felling in humid tropics Soil degradation is greatly responsible for @ 2 billion ha of the 5 billion ha
Land degradation is a global phenomenon, with 78 percent of the degrading land taking place in the non-drylands. Degrading the buffer zone of the drylands increases drylands' vulnerability to ...
Land-degradation impacts are felt by the agricultural industry, and as a result, the people involved in agriculture feel the economic and social effects of land degradation the most. Loss of Economic Value. There is an economic value of the land and its surrounding ecosystem services. The continued availability of productive land and soil would ...
The problem of land degradation is one of the most significant problems in the modern world. More than a half of agricultural land has been degraded to some degree [].In this context, soil and land conservation policy is a priority issue for ensuring food security of the world's population and the goals of sustainable development.
Land Degradation. Article. Land degradation is a process in which the value of the biophysical environment is influenced by a variety of human-induced activities that operate on the land. It is defined as any modification or disturbance to the land that is either detrimental or unwanted. Natural hazards are not considered a cause; nonetheless ...
1. Land is an important natural resource that underpins most development. However, the pressures on land - both anthropogenic and environment-induced - are leading to its degradation. If the rate of land degradation is not reduced, the economies and security of the continent will be highly compromised.
The assessment of land degradation and restoration covers the global status of and trends in land degradation, by region and land cover type; the effect of degradation on biodiversity values, ecosystem services and human well-being; and the state of knowledge, by region and land cover type, of ecosystem restoration extent and options. The assessment aims to enhance the knowledge base for ...
In turn, land-use change is driving land degradation: soil erosion, nutrient depletion, salinity, water scarcity, pollution, disruption of biological cycles, and loss of biodiversity. This is a global issue (UNCED 1992, UNEP 2006), yet there is no authoritative measure of land degradation or its counterpoint - land improvement.
Abstract. Soil degradation by accelerated erosion is a serious problem and will remain so during the 21st century, especially in developing countries of the tropics and subtropics. Yet, its extent, severity, and economic and environmental impacts are debatable. Estimates of global and regional land area affected are tentative and subjective.
Published by Routledge London 2018. ISBN: 978-1-138-68895-7 (hbk) ISBN: 978-1-315-53797-9 (ebk) Chapter 13 Monitoring and assessing land degradation: New approaches pp 249-274. Ian D.L. Foster and ...
reverse land degradation and halt biodiversity loss 103 3.16. Land restoration for achieving SDG 16: Promote peaceful and inclusive societies for sustainable development, provide access to justice for all and build effective, accountable and inclusive institutions at all levels 111 3.17. Land restoration for achieving SDG 17:
tackling land degradation across all land ecosystems. It examines a range of actions that can be taken to reduce land degradation, maintain land productivity and food security, and reverse the adverse impacts of climate change on land degradation, including related policy choices and trade-offs. 5.
Land degradation adversely affects the well-being of approximately 3.2 billion people worldwide and results in a loss of about 10% of annual gross domestic product. Degradation dynamics are often creeping and non-linear, hence the adverse consequences are often not immediately perceived. In consequence, methods for assessing future risks of land degradation and risk reduction strategies are ...
Land degradation, meaning any reduction or loss in the biological or economic productive capacity of the land resource base, is a global problem. Land, water, and forest resources can become degraded due to poor and often short-sighted management. In this context, land degradation neutrality (LDN) is an approach that counterbalances the expected loss of productive land with the restoration of ...
Monitoring land degradation (LD) to improve the measurement of the sustainable development goal (SDG) 15.3.1 indicator ("proportion of land that is degraded over a total land area") is key to ensure a more sustainable future. Current frameworks rely on default medium-resolution remote sensing datasets available to assess LD and cannot ...
Wind erosion is a major natural disaster worldwide, and it is a key problem in western Rajasthan in India. The Analytical Hierarchy Process (AHP), the Geographic Information System (GIS), and remote sensing satellite images are effective tools for modeling and risk assessment of land degradation. The present study aimed to assess and model the land degradation vulnerable (LDV) zones based on ...
Desertification is a type of land degradation in which an already relatively dry land area becomes increasingly arid, degrading productive soil and losing its bodies of water, biodiversity and vegetation cover. It is driven by a combination of factors, including climate change, deforestation, overgrazing and unsustainable agricultural practices.
Additionally, this special issue will scrutinize the role of land degradation in the context of climate change, and how restoration and sustainable management of land resources can mitigate these impacts, supporting the achievement of the United Nations' Sustainable Development Goals, particularly those related to eradicating poverty, achieving ...
DEGRADATION, REHABILITATION, AND CONSERVATION OF SOILS; ... Concentrations of heavy metals and oil products were decreased in different land use zones of the city in the upper horizon of soils disturbed during excavation and reclamation works. ... This study was performed in agreement with state assignment of the Ministry of Science and Higher ...
The Central Valley of California is one of the most prolific agricultural regions in the world. Agriculture is reliant on the conjunctive use of surface-water and groundwater. The lack of available surface-water and land-use changes have led to pumping-induced groundwater-level and storage declines, land subsidence, changes to streamflow and the environment, and the degradation of water quality.
As a heart surgeon, I made major decisions daily guided by what medical science told me. And, right now, scientific evidence increasingly shows that nature - the land, water, plants, and animals ...
Geographic coordinates of Elektrostal, Moscow Oblast, Russia in WGS 84 coordinate system which is a standard in cartography, geodesy, and navigation, including Global Positioning System (GPS). Latitude of Elektrostal, longitude of Elektrostal, elevation above sea level of Elektrostal.
A Dassault Falcon 50EX plane, registered F-GLSA, was destroyed in a take-off accident at Moskva-Vnukovo Airport (VKO), Russia. There were one passenger and three crew members on board. The airplane operated on a flight from Moskva-Vnukovo Airport (VKO) to Paris-Le Bourget Airport (LBG).
21 April 2023 Rosatom obtained a license for the first land-based SMR in Russia. On April 21, Rosenergoatom obtained a license issued by Rostekhnadzor to construct the Yakutsk land-based SMR in the Ust-Yansky District of the Republic of Sakha (Yakutia).
After completing the review of the assignment and transfer fixed filing fee for the proposed rule, the BLM compared the outcome of that review with the inflation adjustments (86 FR 54636 (Oct. 4, 2021)). The review identified that the assignment and transfer fixed filing fee should be $100 in FY2022 based upon the FLPMA factors.