Thank you for visiting nature.com. You are using a browser version with limited support for CSS. To obtain the best experience, we recommend you use a more up to date browser (or turn off compatibility mode in Internet Explorer). In the meantime, to ensure continued support, we are displaying the site without styles and JavaScript.
- View all journals
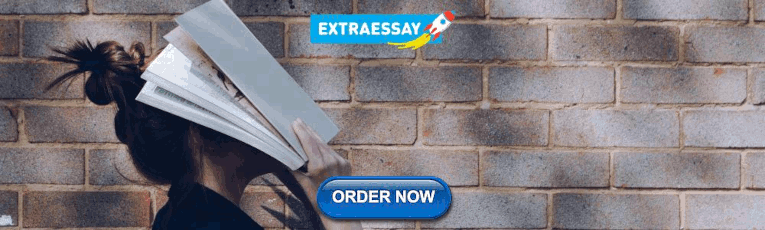
Osteoporosis articles from across Nature Portfolio
Osteoporosis is a metabolic bone disorder characterized by progressive decline of bone mass and bone quality, leading to bone fragility and an increased risk of fracture. Osteoporosis occurs when bone resorption outpaces bone formation during bone remodelling.
Latest Research and Reviews
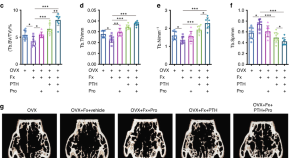
β-Receptor blocker enhances the anabolic effect of PTH after osteoporotic fracture
- Chun-Li Song
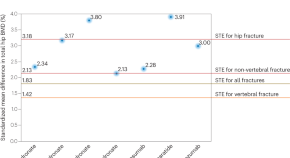
Evidence-Based Guideline for the management of osteoporosis in men
In this Evidence-Based Guideline article, an international multidisciplinary working group of the European Society for Clinical and Economic Aspects of Osteoporosis, Osteoarthritis and Musculoskeletal Diseases presents recommendations for the diagnosis, monitoring and treatment of osteoporosis in men.
- Nicholas R. Fuggle
- Charlotte Beaudart
- Nicholas C. Harvey
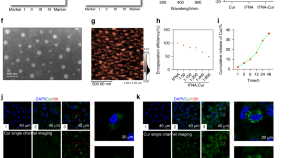
A DNA tetrahedron-based ferroptosis-suppressing nanoparticle: superior delivery of curcumin and alleviation of diabetic osteoporosis
- Zhengwen Cai
- Yunfeng Lin
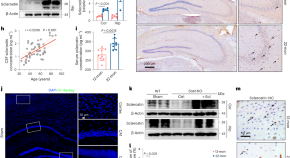
Osteocyte-derived sclerostin impairs cognitive function during ageing and Alzheimer’s disease progression
The authors show that abnormal elevation of osteocyte-derived sclerostin deregulates Wnt–β-catenin signalling in the brain and aggravates cognitive impairment under pathological conditions.
- Tianshu Shi
- Baosheng Guo
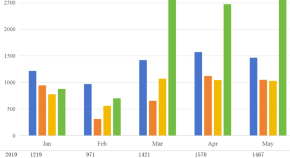
Validation of phenomenon and cross-sectional investigation of predictors for a post-COVID-19 surge of osteoporosis outpatients in China
- Yuehua Zhang
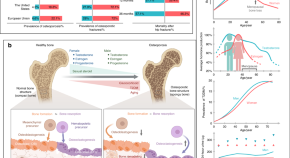
Insights and implications of sexual dimorphism in osteoporosis
- Yuan-Yuan Zhang
- Zhisen Shen
News and Comment
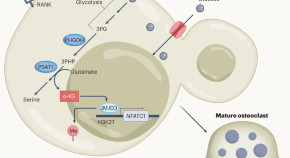
Serine synthesis promotes bone degradation
Bone resorption by osteoclasts requires tight control, as overactivation reduces bone mass and strength. Stegen et al. demonstrate that α-ketoglutarate produced during serine synthesis promotes osteoclast development via metabolic–epigenetic coupling and could be a therapeutic target.
- Ryan C. Riddle
- Gillian M. Choquette
SLC26A2 deficiency, matrix stiffness and mechanotransduction linked in osteoporosis
New research shows that deficiency of the sulfation-related SLC26A2 affects osteocyte formation, and that targeting downstream mediators can ameliorate SLC26A2 -deficient osteoporosis.
- Sarah Onuora
Metal–polyDNA nanoparticles reset the osteoporotic microenvironment
Nanoparticles consisting of long DNA molecules in association with Ca 2+ and PPi 4− ions can modulate the pathological osteoporotic microenvironment and promote bone repair.
- Robert Phillips
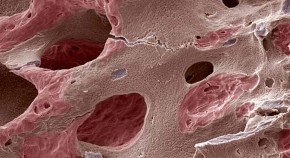
Bone-forming properties of an ascorbic acid derivative
Magnesium ascorbyl phosphate is a stable derivative of ascorbic acid that has now been shown to promote bone formation via stimulation of the CAMKIIα pathway.
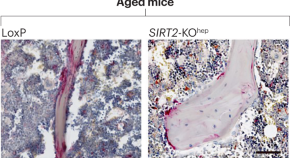
Liver–bone crosstalk implicated in osteoporosis progression
- Olivia Tysoe
New drug formulation reduces bone loss
Quick links.
- Explore articles by subject
- Guide to authors
- Editorial policies

- Skip to main content
- Skip to FDA Search
- Skip to in this section menu
- Skip to footer links

The .gov means it’s official. Federal government websites often end in .gov or .mil. Before sharing sensitive information, make sure you're on a federal government site.
The site is secure. The https:// ensures that you are connecting to the official website and that any information you provide is encrypted and transmitted securely.
U.S. Food and Drug Administration
- Search
- Menu
- News & Events
- FDA Newsroom
- Press Announcements
FDA approves new treatment for osteoporosis in postmenopausal women at high risk of fracture
FDA News Release
The U.S. Food and Drug Administration today approved Evenity (romosozumab-aqqg) to treat osteoporosis in postmenopausal women at high risk of breaking a bone (fracture). These are women with a history of osteoporotic fracture or multiple risk factors for fracture, or those who have failed or are intolerant to other osteoporosis therapies.
More than 10 million people in the U.S. have osteoporosis, which is most common in women who have gone through menopause. People with osteoporosis have weakened bones that are more likely to fracture.
“Today’s approval provides women with postmenopausal osteoporosis who are at high risk of fracture with a new treatment that will reduce this risk,” said Hylton V. Joffe, M.D, M.M.Sc., director of the Center for Drug Evaluation and Research’s Division of Bone, Reproductive and Urologic Products. “But Evenity may increase the risk of heart attack, stroke and cardiovascular death so it’s important to carefully select patients for this therapy, which includes avoiding use in patients who have had a heart attack or stroke within the previous year.”
Evenity is a monoclonal antibody that blocks the effects of the protein sclerostin and works mainly by increasing new bone formation. One dose of Evenity consists of two injections, one immediately following the other, given once a month by a health care professional. The bone forming effect of Evenity wanes after 12 doses so more than 12 doses should not be used. If osteoporosis therapy is needed after the 12 doses, patients should begin an osteoporosis treatment that reduces bone breakdown.
The safety and efficacy of Evenity were demonstrated in two clinical trials involving a total of more than 11,000 women with postmenopausal osteoporosis. In the first trial, one year of treatment with Evenity lowered the risk of a new fracture in the spine (vertebral fracture) by 73% compared to placebo. This benefit was maintained over the second year of the trial when Evenity was followed by one year of denosumab (another osteoporosis therapy) compared to placebo followed by denosumab. In the second trial, one year of treatment with Evenity followed by one year of alendronate (another osteoporosis therapy) reduced the risk of a new vertebral fracture by 50% compared to two years of alendronate alone. Evenity followed by alendronate also reduced the risk of fractures in other bones (nonvertebral fractures) compared to alendronate alone.
Evenity increased the risk of cardiovascular death, heart attack and stroke in the alendronate trial, but not in the placebo trial. Therefore, Evenity contains a boxed warning on its labeling stating that it may increase the risk of heart attack, stroke and cardiovascular death and should not be used in patients who have had a heart attack or stroke within the previous year. Health care professionals should also consider whether the benefits of Evenity outweigh its risks in those with other risk factors for heart disease and should discontinue Evenity in any patient who experiences a heart attack or stroke during treatment.
Common side effects of Evenity included joint pain and headache. Injection site reactions were also observed.
The FDA granted the approval of Evenity to Amgen.
The FDA, an agency within the U.S. Department of Health and Human Services, protects the public health by assuring the safety, effectiveness, and security of human and veterinary drugs, vaccines and other biological products for human use, and medical devices. The agency is also responsible for the safety and security of our nation’s food supply, cosmetics, dietary supplements, products that give off electronic radiation, and for regulating tobacco products.
Related Information
- Osteoporosis: Common treatments
- How Long Should You Take Certain Osteoporosis Drugs?

Bone-loss discovery points to new treatment for osteoporosis
A new discovery about osteoporosis suggests a potential treatment target for that brittle-bone disease and for bone loss from rheumatoid arthritis.
The findings from University of Virginia School of Medicine researchers and their collaborators help explain why specialized bone cells called osteoclasts begin to break down more bone than the body replaces. With more research, scientists one day may be able to target that underlying cause to prevent or treat bone loss.
The discovery also suggests an answer for why some previous attempts to develop osteoporosis treatments produced disappointing results.
"Bone degradation and subsequent repair are fine-tuned through complex interactions between the cells that degrade the bone, osteoclasts, and those that produce new bone matrix. Simple elimination of osteoclasts is, therefore, not always the best approach to treat pathologic bone loss. Instead, we found a 'signaling node' in osteoclasts that regulates their function in degrading the bone but doesn't reduce osteoclast numbers," said researcher Sanja Arandjelovic, PhD, of UVA's Department of Medicine and UVA's Carter Immunology Center.
Researcher Kodi Ravichandran, PhD, chairman of UVA's Department of Microbiology, Immunology and Cancer Biology and director of UVA's Center for Cell Clearance, noted the potential of the findings to inform efforts to develop better treatments for osteoporosis: "In this study," he said, "we identified previously unappreciated factors that contribute to osteoclast function that is truly exciting and opens up new avenues to pursue."
Understanding Bone Loss
Osteoporosis affects more than 200 million people around the world, and it causes bone fractures in 1 in 3 women and 1 in 5 men over age 50. Bone loss is also seen in rheumatoid arthritis, a painful inflammatory condition that affects up to 1% of people, including more than 1.3 million Americans.
Scientists are eager to understand what causes this bone loss, and to develop new ways to treat and prevent it. Arandjelovic, Ravichandran and their collaborators have found an important contributor, a cellular protein called ELMO1. This protein, they found, promotes the activity of the bone-removing osteoclasts. While osteoclasts may seem like 'bad guys' because they remove bone, they are critical for bone health, as they normally remove just enough to stimulate new bone growth. The problem arises when the osteoclasts become too aggressive and remove more bone than the body makes. Then bone density suffers and bones grow weaker.
This excessive bone degradation is likely influenced by genetic factors, the researchers say. They note that many of the genes and proteins linked to ELMO1 have been previously associated with bone disorders and osteoclast function.
Treating Osteoporosis and Rheumatoid Arthritis
Encouragingly, the researchers were able to prevent bone loss in lab mice by blocking ELMO1, including in two different models of rheumatoid arthritis. That suggests clinicians may be able to target the protein in people as a way to treat or prevent bone loss caused by osteoporosis and RA, the researchers say.
They note that prior efforts to treat osteoporosis by targeting osteoclasts have had only mixed success, and they offer a potential explanation for why: Osteoclasts not only remove bone but play a role in calling in other cells to do bone replacement. As such, targeting ELMO1 may offer a better option than simply waging war on the osteoclasts.
"We used a peptide to target ELMO1 activity and were able to inhibit degradation of the bone matrix in cultured osteoclasts without affecting their numbers," Ravichandran said. "We hope that these new osteoclast regulators identified in our study can be developed into future treatments for conditions of excessive bone loss such as osteoporosis and arthritis."
- Osteoporosis
- Bone and Spine
- Women's Health
- Medical Topics
- Bone marrow
- Periodontal disease
- Rheumatoid arthritis
- Osteoarthritis
- Bone fracture
Story Source:
Materials provided by University of Virginia Health System . Note: Content may be edited for style and length.
Journal Reference :
- Sanja Arandjelovic, Justin S. A. Perry, Ming Zhou, Adam Ceroi, Igor Smirnov, Scott F. Walk, Laura S. Shankman, Isabelle Cambré, Suna Onengut-Gumuscu, Dirk Elewaut, Thomas P. Conrads, Kodi S. Ravichandran. ELMO1 signaling is a promoter of osteoclast function and bone loss . Nature Communications , 2021; 12 (1) DOI: 10.1038/s41467-021-25239-6
Cite This Page :
Explore More
- Illuminating Oxygen's Journey in the Brain
- DNA Study IDs Descendants of George Washington
- Heart Disease Risk: More Than One Drink a Day
- Unlocking Supernova Stardust Secrets
- Why Do Some Memories Become Longterm?
- Cell Division Quality Control 'Stopwatch'
- What Controls Sun's Differential Rotation?
- Robot, Can You Say 'Cheese'?
- Researchers Turn Back the Clock On Cancer Cells
- Making Long-Term Memories: Nerve-Cell Damage
Trending Topics
Strange & offbeat.
- Alzheimer's disease & dementia
- Arthritis & Rheumatism
- Attention deficit disorders
- Autism spectrum disorders
- Biomedical technology
- Diseases, Conditions, Syndromes
- Endocrinology & Metabolism
- Gastroenterology
- Gerontology & Geriatrics
- Health informatics
- Inflammatory disorders
- Medical economics
- Medical research
- Medications
- Neuroscience
- Obstetrics & gynaecology
- Oncology & Cancer
- Ophthalmology
- Overweight & Obesity
- Parkinson's & Movement disorders
- Psychology & Psychiatry
- Radiology & Imaging
- Sleep disorders
- Sports medicine & Kinesiology
- Vaccination
- Breast cancer
- Cardiovascular disease
- Chronic obstructive pulmonary disease
- Colon cancer
- Coronary artery disease
- Heart attack
- Heart disease
- High blood pressure
- Kidney disease
- Lung cancer
- Multiple sclerosis
- Myocardial infarction
- Ovarian cancer
- Post traumatic stress disorder
- Rheumatoid arthritis
- Schizophrenia
- Skin cancer
- Type 2 diabetes
- Full List »
share this!
March 25, 2024
This article has been reviewed according to Science X's editorial process and policies . Editors have highlighted the following attributes while ensuring the content's credibility:
fact-checked
trusted source
New evidence-based guideline for the management of osteoporosis in men
by International Osteoporosis Foundation
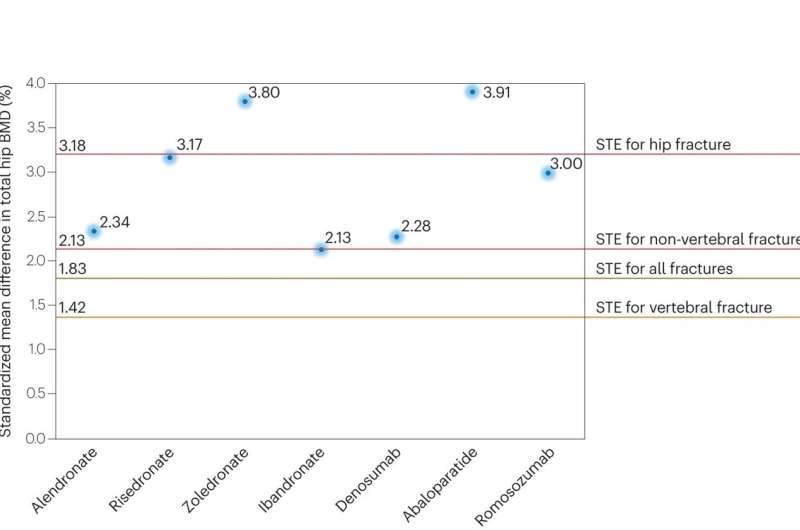
Worldwide, it is estimated that one in five men over the age of 50 years will experience an osteoporotic fracture in their remaining lifetime, and the number of hip fractures in men is expected to rise by approximately 310% between 1990 and 2050. Despite its great burden among older men, osteoporosis is still often viewed as a 'woman's' disease, and underdiagnosis and undertreatment of the condition in men are even more prevalent than in women.
In response, an international multidisciplinary working group of the European Society for Clinical and Economic Aspects of Osteoporosis, Osteoarthritis and Musculoskeletal Diseases (ESCEO) has now issued GRADE-assessed recommendations for the diagnosis, monitoring, and treatment of osteoporosis in men.
Professor Jean-Yves Reginster, senior author and President of ESCEO, stated, "It's important to recognize that osteoporosis in men carries substantial morbidity and mortality, with rates comparable to or even exceeding those in women with the condition."
"The ESCEO international working group was convened to provide new management recommendations that are informed by the latest developments in research and up-to-date expert opinion related to diagnostic and screening approaches for osteoporosis and its associated high fracture risk in men."
The Working Group recommendations cover disease burden, approaches to fracture risk assessment in men, including appropriate interpretation of bone densitometry and absolute fracture risk, thresholds for treatment, and interventions that can be used therapeutically together with their health economic evaluation.
The guidance also notes the need for more research with future work specifically addressing the efficacy of anti-osteoporosis medications, including denosumab and bone-forming therapies.
Among the key recommendations and guidelines, the following may be particularly useful for clinicians:
- A female reference database should be used for the densitometric diagnosis of osteoporosis in men.
- FRAX is the appropriate tool for the assessment of fracture risk and as the basis for setting intervention thresholds in men with osteoporosis.
- FRAX-based intervention thresholds should be age-dependent in men with osteoporosis.
- Trabecular bone score, used with BMD and FRAX probability, provides useful information for fracture risk assessment in men.
- All men with a prior fragility fracture should be considered for treatment with anti-osteoporosis medications.
- The anti-osteoporosis treatment regimen in men should be adapted to an individual's baseline fracture risk.
- Vitamin D and calcium repletion should be ensured in all men above the age of 65 years.
- Oral bisphosphonates (alendronate or risedronate) are first-line treatments for men at a high risk of fracture.
- Denosumab or zoledronate are second-line treatments for men at a high risk of fracture.
- A sequential therapy starting with a bone-forming agent followed by an anti-resorptive agent should be considered for men at a very high risk of fracture.
- Biochemical markers of bone turnover are the appropriate tool to assess adherence to anti-resorptive therapy in men.
- Bone-forming agents when given as first-line treatment in men at a very high risk of fracture, should be used in accordance with the recommendations of the regulatory authorities.
- Physical exercise and a balanced diet should be recommended to all men with osteoporosis.
- Serum total testosterone should be assessed as part of the pre-treatment assessment of men with osteoporosis.
- Appropriate hormone replacement therapy should be considered in men with low levels of total or free serum testosterone.
- Based on available BMD data, abaloparatide is considered an appropriate first-line treatment for men with osteoporosis at a very high risk of osteoporotic fracture.
Professor Nicholas Harvey, senior author and chairman of the International Osteoporosis Foundation (IOF) Committee of Scientific Advisors, noted, "We hope that these guidelines will assist clinicians in their clinical practice and encourage them to be proactive in managing osteoporosis in their male patients."
"Following an approach similar to that advocated for women with osteoporosis, we recommend the use of oral anti-resorptive agents as first-line agents in men at a high risk of fracture and the use of bone-forming agents followed sequentially by anti-resorptive agents in men at a very high risk of fracture."
IOF CEO Dr. Philippe Halbout concluded, "Osteoporosis in men poses an enormous global burden, and must be urgently addressed by health care providers as well as health authorities. As the world's largest global organization in the osteoporosis arena, IOF welcomes the publication of this important new guideline which we hope will contribute to better patient care and to reducing the devastating consequences of osteoporosis in older men around the world."
The work is published in the journal Nature Reviews Rheumatology .
Explore further
Feedback to editors

Person is diagnosed with bird flu after being in contact with cows in Texas
46 minutes ago

'Pathogen prospecting': Mosquito researchers track malaria's history by examining its epidemiology
49 minutes ago

Pilot study shows ketogenic diet improves severe mental illness
50 minutes ago

Chatbot outperforms physicians in clinical reasoning, but also underperforms against residents on many occasions

Reducing late-night alcohol sales curbed all violent crimes by 23% annually in a Baltimore neighborhood: Study

Scientists pioneer immunotherapy technique for autoimmune diseases

Researchers develop more broadly protective coronavirus vaccine

Scientists discover speed of visual perception ranges widely in humans
2 hours ago

Move more, sleep better: Study finds physical activity lengthens REM latency
3 hours ago

Siris tree leaves hold promise for stopping spread of breast cancer cells, say researchers
4 hours ago
Related Stories

Women with osteoporosis want to know their fracture risk
Jan 1, 2024

A new aid to osteoporosis and fracture risk patient discussion for primary care providers
Jun 1, 2022

Update on use of trabecular bone score (TBS) in clinical practice
Jul 21, 2023

New position paper offers practical guidance for osteoporosis management
Nov 20, 2019
Updated European guidance for the diagnosis and management of osteoporosis in women
Nov 1, 2018

Study reveals gaps in fracture risk communication and patients' preferences for visual representation
Nov 20, 2023
Recommended for you

Psychological care delivered by phone can help combat loneliness and depression, study finds
Mar 29, 2024

Study suggests maintaining optimism contributes to better mobility in women as they grow older
Mar 28, 2024

Patients with delirium more likely to develop dementia, finds study

Old immune systems revitalized in mouse study, may improve vaccine response in the elderly
Mar 27, 2024

Couples with similar drinking habits may live longer

Chronic musculoskeletal pain may accelerate brain aging
Mar 26, 2024
Let us know if there is a problem with our content
Use this form if you have come across a typo, inaccuracy or would like to send an edit request for the content on this page. For general inquiries, please use our contact form . For general feedback, use the public comments section below (please adhere to guidelines ).
Please select the most appropriate category to facilitate processing of your request
Thank you for taking time to provide your feedback to the editors.
Your feedback is important to us. However, we do not guarantee individual replies due to the high volume of messages.
E-mail the story
Your email address is used only to let the recipient know who sent the email. Neither your address nor the recipient's address will be used for any other purpose. The information you enter will appear in your e-mail message and is not retained by Medical Xpress in any form.
Newsletter sign up
Get weekly and/or daily updates delivered to your inbox. You can unsubscribe at any time and we'll never share your details to third parties.
More information Privacy policy
Donate and enjoy an ad-free experience
We keep our content available to everyone. Consider supporting Science X's mission by getting a premium account.
E-mail newsletter
Recent Progresses in the Treatment of Osteoporosis
Affiliations.
- 1 Department of Rheumatology, The First Affiliated Hospital of Zhengzhou University, Zhengzhou, China.
- 2 Department of Orthopaedics, The First Affiliated Hospital of Zhengzhou University, Zhengzhou, China.
- 3 Department of Magnetic Resonance Imaging, Henan Key Laboratory of Functional Magnetic Resonance Imaging and Molecular Imaging, The First Affiliated Hospital of Zhengzhou University, Zhengzhou, China.
- PMID: 34366868
- PMCID: PMC8339209
- DOI: 10.3389/fphar.2021.717065
Osteoporosis (OP) is a chronic bone disease characterized by aberrant microstructure and macrostructure of bone, leading to reduced bone mass and increased risk of fragile fractures. Anti-resorptive drugs, especially, bisphosphonates, are currently the treatment of choice in most developing countries. However, they do have limitations and adverse effects, which, to some extent, helped the development of anabolic drugs such as teriparatide and romosozumab. In patients with high or very high risk for fracture, sequential or combined therapies may be considered with the initial drugs being anabolic agents. Great endeavors have been made to find next generation drugs with maximal efficacy and minimal toxicity, and improved understanding of the role of different signaling pathways and their crosstalk in the pathogenesis of OP may help achieve this goal. Our review focused on recent progress with regards to the drug development by modification of Wnt pathway, while other pathways/molecules were also discussed briefly. In addition, new observations made in recent years in bone biology were summarized and discussed for the treatment of OP.
Keywords: anabolic drugs; antiresorptive drugs; bone formation; osteoporosis; wnt signaling pathway.
Copyright © 2021 Li, He, Xie, Li, Zhang, Li and Li.
Publication types

New discovery leads the way to reverse osteoporosis — with a pill
By Angela Nicoletti
January 23, 2023 at 11:42am
Reversing osteoporosis could one day be as easy as taking a pill.
A team of FIU drug development scientists found a possible new way to counteract the effects of the disease that turns bones into honeycomb-like structures — so fragile even a cough can cause a fracture or break. The discovery, recently published in Communications Biology , is the first step toward cheaper, effective, easy-to-take treatments for osteoporosis and other diseases associated with bone loss.
Researchers from FIU’s Herbert Wertheim College of Medicine — along with a collaborative team from the National Center for Advancing Translational Sciences ( NCATS), part of the National Institutes of Health (NIH), and University of Arkansas for Medical Sciences — identified a method that can be taken orally and helps bone-producing cells make more bone.
“Our experiments in the lab showed small molecule activators delivered orally improve bone density, an exciting discovery that could lead to a new treatment for osteoporosis,” said study author Alexander Agoulnik , FIU professor and interim chair of the Department of Human and Molecular Genetics .
Bones are essentially always under renovation. Cells break down and reform bone around the clock. Bone-making cells build bone tissue, while bone-eating ones reabsorb it to keep bones from getting too big or bulky. It’s a delicate dance. And it goes awry during osteoporosis when bone-making cells slow down and bone-eating cells keep at a steady pace.
To help bone-producing cells keep up, the researchers focused on a specific target. A hormone receptor — relaxin family peptide receptor 2 (RXFP2), known to play a role in the formation of reproductive organs.
“When we first screened patients with cryptorchidism, or undescended testes, we found in some of them this receptor was mutated,” Agoulnik said. “Then, colleagues from the University of Padova, Italy, surprisingly discovered that a significant portion of these men also happened to have osteoporosis.”
The team suspected the receptor also had something to do with bone development. Further research revealed it did.
The team first had to pinpoint the right chemical compound to activate the receptor. The NCATS team used robots to screen for small molecules, among more than 80,000 different compounds. Chemists then tested hundreds more variations until they found the right match. It was like a key fitting a lock. When they tested it on mouse models in the lab, they saw an improvement in bone density.
"I chose FIU because FIU has a very diverse international community." Maria Esteban Lopez is the FIU Medicine alum who helped make the recent discovery that could lead the way to reverse osteoporosis — with a pill. Read more about it: https://t.co/bpHKX3Auiu pic.twitter.com/qo7PU1hqsp — FIU (@FIU) January 23, 2023
“This opens up a new area of study to allow for clinical application to prevent or reverse osteoporosis,” said Maria Esteban Lopez, who worked with Agoulnik on this research as an FIU biomedical sciences Ph.D. candidate. “This was an exciting opportunity. I was able to work with phenomenal scientists across various institutions and see how the impact of our work could lead to meaningful therapeutic breakthroughs.”
Researchers know a new treatment could be life-changing for millions of people, and look forward to the next phase of research.
“Without collaboration, we would never have found the link between our genes and bone development. And the collaboration with NIH was crucial because they are the best in the field to look for the right compounds,” Agoulnik said. “The main ingredient for success in science is collaboration.”
This research was supported by the National Institute of Arthritis and Musculoskeletal and Skin Diseases (NIAMS) at the NIH.
Media contact:
Angela Nicoletti [email protected] 305-348-0272
- Review Letter
- Open access
- Published: 04 September 2022
Osteoporosis pathogenesis and treatment: existing and emerging avenues
- Bo Liang 1 ,
- George Burley ORCID: orcid.org/0000-0002-2560-3604 2 ,
- Shu Lin 2 , 3 na1 &
- Yan-Chuan Shi ORCID: orcid.org/0000-0002-8368-6735 2 , 3 , 4 na1
Cellular & Molecular Biology Letters volume 27 , Article number: 72 ( 2022 ) Cite this article
11k Accesses
46 Citations
1 Altmetric
Metrics details
Osteoporotic fractures lead to increased disability and mortality in the elderly population. With the rapid increase in the aging population around the globe, more effective treatments for osteoporosis and osteoporotic fractures are urgently required. The underlying molecular mechanisms of osteoporosis are believed to be due to the increased activity of osteoclasts, decreased activity of osteoblasts, or both, which leads to an imbalance in the bone remodeling process with accelerated bone resorption and attenuated bone formation. Currently, the available clinical treatments for osteoporosis have mostly focused on factors influencing bone remodeling; however, they have their own limitations and side effects. Recently, cytokine immunotherapy, gene therapy, and stem cell therapy have become new approaches for the treatment of various diseases. This article reviews the latest research on bone remodeling mechanisms, as well as how this underpins current and potential novel treatments for osteoporosis.
Introduction
In the face of an increasingly aging population, osteoporosis (OP) is becoming one of the most common diseases worldwide. At the time of the last US Census in 2010, the overall prevalence of osteoporosis in adults aged 50 years and older was approximately 10.2 million. The prevalence was significantly higher in women (16.5%) than in men (5.1%) [ 1 ]. Osteoporosis is characterized by deteriorated bone strength and a subsequent increase in fracture risk [ 2 ]. Osteoporotic fractures, or fragility fractures, are responsible for significant reductions in quality of life, as well as increased social and economic burdens at an individual and population level. This is particularly true for hip fractures; within a year of sustaining a hip fracture for those aged over 50 years, approximately 20% of patients will be dead, and nearly 50% of patients will be disabled [ 3 ].
The clinical diagnosis of osteoporosis is based mainly on bone mineral density (BMD), measured using dual-energy X-ray absorptiometry (DEXA), and/or the occurrence of fragility fractures [ 2 ]. The fracture risk prediction tool (FRAX), recommended by the World Health Organization (WHO), can be used to evaluate the incidence of osteoporotic fractures. This prediction tool includes major risk factors for osteoporotic fracture: age, sex, body mass index (BMI), fracture history, smoking, glucocorticoid medication history, rheumatoid arthritis, diseases that can cause secondary osteoporosis, and BMD [ 4 ]. At present, the treatment of osteoporosis is based on its pathogenesis, which is studied at different stages of disease development.
Bone consists of dense outer cortical bone and spongy inner cancellous bone, both having distinct properties that work together to maintain bone strength. They are made up of cells, including osteocytes, osteoclasts, osteoblasts and stem cells, and bone matrix, which is composed of calcium, phosphorus, inorganic salts and bone collagen. Osteoclasts resorb bone, whereas osteoblasts form new bone. The antagonistic actions of these two cell types occur constantly in the body in order to maintain bone health and structural integrity of the skeleton. This process is termed bone remodeling or bone turnover [ 5 ]. Any factors that decrease the activity of osteoblasts and/or increase the activity of osteoclasts will result in greater bone resorption than bone formation. This imbalance in bone remodeling also induces the destruction of bone microstructure, especially the structural destruction of cancellous bone, which leads to a decrease in bone strength and subsequent fragility fractures.
By exploring the underlying molecular mechanisms of imbalances in bone remodeling, novel osteoporosis treatments have been developed. Bisphosphonates, acting to inhibit bone resorption, are one such example, whose clinical application has brought revolutionary changes to osteoporosis treatment [ 6 ]. Another example is denosumab, a monoclonal antibody targeting the nuclear factor kappa B (NF-κB) ligand activated receptor (RANKL), serving to slow bone breakdown [ 7 ]. Its clinical application in recent years displays the successful application of cytokine immunotherapy in osteoporosis treatment [ 8 ]. However, both bisphosphonates and denosumab still have limitations and side effects, such as mandibular osteonecrosis and atypical femoral fractures [ 9 ]. Estrogen replacement therapy for postmenopausal women has been shown to be another effective osteoporosis therapy. Menopause, typified by reducing estrogen levels, is an important risk factor for osteoporosis. In 2020, the American Association of Clinical Endocrinologists (AACE) issued the “Guidelines for the Diagnosis and Treatment of Postmenopausal Osteoporosis,” in which intervention and treatment measures have been proposed on the basis of the etiology of postmenopausal osteoporosis [ 10 ]. Studies have shown that estrogen can affect bone remodeling by inhibiting osteoclast activity [ 11 ]. Although estrogen replacement therapy can effectively reduce menopause-associated osteoporosis risk, it is associated with life-threatening complications such as venous thrombosis and increased tumor development [ 12 ]. In light of the shortcomings of current therapies, it is necessary to continue studying the molecular mechanisms of osteoporosis in order to identify further new treatments. Different micro-RNAs (miRNAs) have been found to play important roles in the regulation of osteoblast and osteoclast activities [ 13 ]. Thus, miRNAs could be used as a potential biomarker and therapeutic target for osteoporosis. It is also possible to treat osteoporosis by harnessing the osteogenic differentiation ability of stem cells and their paracrine role in regulating cell function [ 14 ]. Thus far, stem cells have been used to treat osteoporosis in both rabbit and rat models [ 15 ], meaning the development of stem cell therapy in the clinical setting is imminent.
This article briefly summarizes the updates in the molecular basis of bone remodeling and the currently available treatment strategies for osteoporosis. More importantly, emerging new research directions are described, namely miRNAs, stem cells, bone marrow adipocytes, nerves and endothelium, gut microbiota, and bone targeting technologies, to shed light on future therapeutic avenues for this burdensome disease.
The regulation of bone remodeling in health and osteoporosis
Osteoporosis results from an imbalance of normal bone remodeling, such that bone resorption is favored over bone formation. Human bones are stimulated by body weight, muscle traction, and high-intensity exercise. Over time, bones are damaged and degraded. Bone remodeling starts with bone resorption and ends with bone formation (Fig. 1 ). It is an essential process for maintaining mechanical strength, structural integrity, and mineralization by replacing old and damaged bone with new bone. However, the exact initial mechanisms underlying remodeling are yet to be fully elucidated. It is known that the process occurs in response to a number of factors, including hormone signals, paracrine and autocrine factors, and the physical pressure of mechanical loading [ 16 ]. Additionally, a range of systems —endocrine, immune, nervous, and more—are involved in the regulation of bone remodeling [ 17 ]. Environmental and genetic factors further influence this process; menopause, low BMI, white or Asian background, lack of sunshine, low exercise, malnutrition, disease, and certain drugs lead to bone microstructure damage and osteoporosis [ 18 ]. Although the exact mechanisms that initiate osteoporosis are yet to be fully elucidated, the signaling pathways that regulate bone resorption and formation have been extensively described. These are briefly outlined below. This is significant to note as most of the existing medications under development have focused on targeting such pathways, which mainly comprise mechanisms to control osteoclast and osteoblast action [ 19 ].
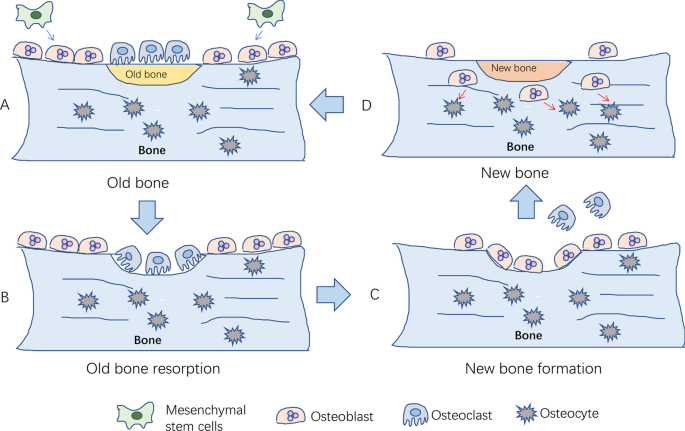
The process of bone remodeling under physiological conditions. A Local bone degenerates into old bone. Mesenchymal stem cells differentiate into osteoblasts; B osteoclasts migrate to the surface of old bone for bone resorption; C osteoclasts leave the surface after the old bone is absorbed, and then osteoblasts migrate to the surface for bone formation; D new bone replaces old bone to maintain bone quality, strength, and mass. After bone formation, osteoblasts differentiate into osteocytes
Osteoclast differentiation and regulation of bone resorption
Osteoclasts are the primary functional cells involved in bone resorption. They are granulocyte–macrophage colonies in the mononuclear macrophage system, formed by the fusion of monocyte precursors under the action of various factors secreted by bone marrow stromal cells [ 20 ]. Drawn by the action of chemokines, osteoclast precursors enter circulation and reach bone tissue in the absorptive state. These precursors are then induced to differentiate into osteoclasts by granulocyte macrophage colony-stimulating factor (GM-CSF) and RANKL. Mature osteoclasts then cover the surface of the absorbed bone tissue and release osteolysis-related enzymes for bone resorption [ 21 ]. It has been well documented that various factors affect bone resorption, including hormones, cytokines, and noncoding RNAs, by acting on signaling pathways in osteoclast differentiation. Among these signaling pathways, the RANKL/RANK/OPG and IL-1/TNF-α pathways are known to be critical for osteoclastogenesis, described below (Fig. 2 ).
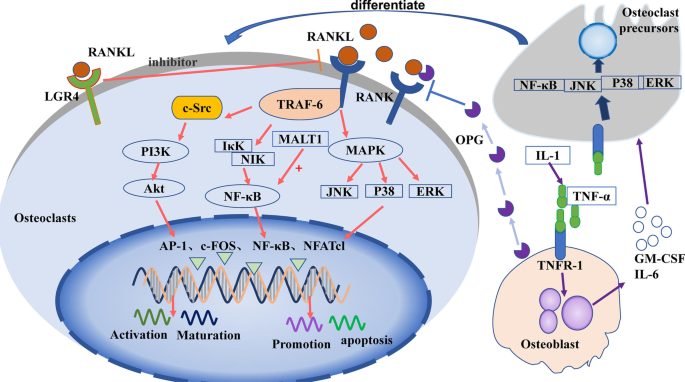
Signaling pathways in the control of osteoclast differentiation and maturation
RANKL/RANK/OPG signaling pathway
The RANKL/RANK/OPG signaling pathway is one of the most studied signaling pathways in bone homeostasis. It is essential to normal physiology, functioning to potently promote osteoclast differentiation and activity [ 22 ]. After being secreted by osteocytes, RANKL binds to the RANKL-specific receptor (RANK) on osteoclasts to upregulate their differentiation and activation [ 23 ]. Osteoprotegerin (OPG), a decoy receptor, is mainly produced by osteoblasts, and competes with RANKL to negatively regulate osteoclast differentiation [ 24 ].
RANKL binds to RANK to form a trimer, which then binds molecules to recruit tumor necrosis factor receptor-related factor-6 (TRAF-6). TRAF-6 passes through NF-κB inhibitor-κ-binding kinase (IκK) and NF-κB-induced kinase (NIK), causing them to activate NF-κB, which regulates osteoclast maturation, differentiation, or apoptosis [ 25 ]. TRAF-6 also activates c-Src [ 26 ], which stimulates phosphatidylinositol 3-kinase (PI3K). PI3K activates protein kinase B (PKB, Akt), which subsequently regulates osteoclast differentiation [ 27 ]. Additionally, RANKL/RANK activates the mitogen-activated protein kinase (MAPK) signaling pathway via extracellular regulated protein kinases (ERK1/2), c-Jun N-terminal kinase (JNK), or P38MAPK. The MAPK pathway results in the activation of transcription factors c-fos, activator protein-1 (AP-1), and nuclear factor of activated T cells-1 (NFATc1) [ 28 ], which then regulate the expression of matrix metalloproteinases (MMPs) [ 20 ] to stimulate the differentiation of osteoclast precursors into osteoclasts [ 29 ]. Recent studies suggest that protein phosphatase 2A (PP2A) promotes the expression of RANKL [ 30 ]. In addition, the leucine-rich G-protein-coupled receptor 4 (LGR4) was recently identified as another receptor of RANKL [ 31 ]. This is thought to competitively bind RANKL, thereby inhibiting the classical RANKL–RANK signal transduction pathway during osteoclast differentiation.
IL-1/TNF-α signaling pathway
IL-1 can induce tumor necrosis factor-α (TNF-α) to stimulate osteoblasts to produce granulocyte macrophage colony-stimulating factor (GM-CSF) and IL-6 [ 32 ], and induce osteoclast precursors to differentiate into osteoclasts [ 33 ]. TNF-α can also bind to TNF receptor-1 (TNFR-1) of osteoclast precursors, activate NF-κB, JNK, p38, or ERK, and promote the differentiation of osteoclast precursors into osteoclasts [ 34 ].
MALT1 signaling pathway
Mucosa-associated lymphoid tissue lymphoma translocation factor 1 (MALT1) regulates the NF-κB–NFATc1 signaling pathway and promotes osteoclast activation [ 35 ]. Following studies have shown that inhibitors of MALT1 inhibit NF-κB in osteoclasts, thereby strongly inhibiting the expression of NFATc1 and reducing osteoclast differentiation [ 36 ].
In osteoclasts, RANKL binds to RANK, then activates PI3K/Akt, NF-κB, or MAPK signaling via the recruitment protein TRAF-6, further activating transcription factors, such as AP-1, c-fos NF-κB, and NFATc1 to regulate osteoclast function. LGR4 inhibits the RANKL/RANK signaling pathway by binding to RANK. Osteoblasts secrete OPG to inhibit RANKL signaling and release GM-CSF or IL-6 to promote the differentiation of osteoclast precursors after induction with IL-1 or TNF-α.
Signal pathways controlling osteoblast proliferation and differentiation
As with osteoclasts in bone resorption, osteoblasts are the major functional cells of bone formation. The precursor cells of osteoblasts are multipotent bone marrow mesenchymal stem cells (BM-MSCs), capable of several different cell lineages including osteoblasts, adipocytes, and chondrocytes [ 37 ]. After being stimulated to differentiate into osteoblasts, they are deposited on the bone surfaces. Here, they encourage bone formation and strength by synthesizing and secreting collagen, and promoting the mineralization of inorganic phosphorus and calcium ions to form hydroxyapatite. Osteoblasts may remain as bone-lining cells, or they can also be embedded in the bone matrix, at which point they become osteocytes. After repeating this process of osteoblast deposition and embedding multiple times, a new bone matrix is formed [ 38 ]. In terms of stem cell osteogenic differentiation and osteoblast activation, the most studied signaling pathways include the Wnt/β-catenin, BMP–Smad, Hedgehog, and Notch signaling pathways (Fig. 3 ).
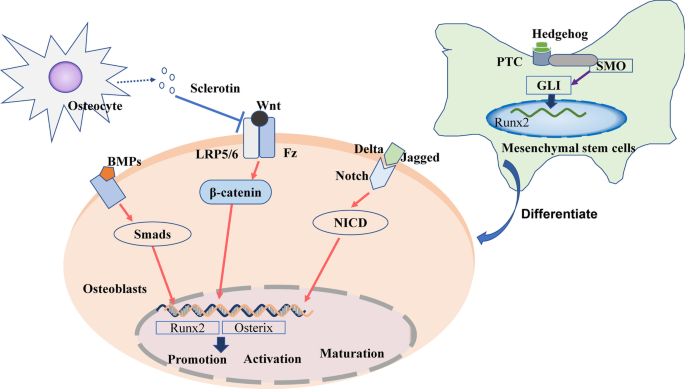
Signaling pathways regulating osteoblast differentiation and maturation
Wnt/β-catenin signaling pathway
The Wnt signaling pathway includes both canonical and noncanonical pathways [ 39 ]. Of these two pathways, the canonical Wnt signaling pathway has been shown to play a particularly important role in osteoblastic bone remodeling [ 40 ]. The binding of Wnt protein in osteoblasts to low-density lipoprotein receptor-related proteins (LRP5/6) and Frizzled (Fz) receptors, located on the osteoblast membrane, promotes the stabilization of intracellular β-catenin [ 41 ]. β-Catenin can then translocate into the nucleus and regulate the expression of osterix and Runt-related transcription factor 2 (Runx2). These are key bone-specific transcription factors for osteogenesis [ 42 ], which thereby influence osteoblast activity [ 43 ].
BMP–smad signaling pathway
Bone morphogenetic proteins (BMPs) are important members of the transforming growth factor-β superfamily [ 44 ]. BMP2, 4, 7, and 9 play important roles in the differentiation of osteoblasts [ 44 ]. They bind to specific receptors on the cell membrane to phosphorylate downstream Smad proteins [ 45 ] (such as Smad1 and 5) and then further activate transcription factors [ 46 ], including Runx2 and osterix [ 47 ].
Hedgehog signaling pathway
The Hedgehog (Hh) signaling pathway is composed of Hh-corresponding ligands (IHH, Shh, DHH), receptors (Patched PTC, SMO), and intracellular signaling molecules (e.g., GLIs) [ 48 ]. After Hh binds to the PTC and SMO receptors on mesenchymal stem cell (MSC) membranes [ 49 ], it activates GLIs, which are translocated into the nucleus to upregulate the expression of the downstream target Runx2 [ 50 ]. This results in MSC differentiation into osteoblasts instead of adipocytes [ 51 ].
Notch signaling pathway
The role of the Notch signaling pathway in skeletal metabolism is not always consistent. Jagged and delta-like proteins, such as Notch ligands, have been found to bind Notch and promote the translocation of the intracellular domain of Notch (NICD) into the nucleus, thus promoting osteoblast differentiation in vitro [ 52 ]. However, some studies have shown that NOTCH1 inhibits osteoclastogenesis, NOTCH2 enhances osteoclast differentiation [ 53 ], and NOTCH3 is the main signal of Notch signaling in osteoblasts [ 54 ]. As such, the role of the Notch signaling pathway in bone remodeling requires further elucidation.
In bone-forming osteoblasts, Wnt binds to the LRP5/6 or Fz receptors, induces β-catenin translocation into the nucleus, and activates the expression of osterix and Runx2 to regulate the promotion, activation, and maturation of osteoblasts. BMPs promote Smad phosphorylation to activate the expression of osterix and Runx2. Jagged and delta-like proteins bind to Notch, induce NICD translocation into the nucleus, and activate the expression of osterix and Runx2. Sclerotin secreted by osteocytes inhibits Wnt binding to osteoblasts. In bone marrow mesenchymal stem cells (BM-MSCs), Hh binds to the PTC and SMO receptors and activates GLIs that translocate into the nucleus to upregulate the expression of Runx2, promoting MSC differentiation into osteoblasts.
Nutrients and known regulatory factors in modulating bone remodeling
The occurrence and development of osteoporosis is closely related to factors that regulate bone remodeling such as calcium, vitamin D, estrogen, and parathyroid hormone (PTH). As such, these factors have formed the basis for current pharmacological treatment strategies. The recent emergence of drugs targeting cytokines, such as RANKL and OPG, which regulate osteoclast activity, indicates how osteoporotic therapy has entered a new frontier steeped in molecular biology.
Calcium and vitamin D
Ninety-nine percent of the body’s calcium is stored in the bones. Sufficient calcium intake is essential for maintaining bone mass and strength. This is also dependent on sufficient intake and activation of vitamin D, which promotes effective absorption of intestinal calcium. Active vitamin D [1,25 (OH)2D3] also directly promotes bone health by binding to vitamin D receptors (VDRs) in bone cells to regulate bone remodeling [ 55 ]. To be converted into its active form [ 56 ], it is hydroxylated twice, first in the liver and then in the kidneys. 1,25(OH)2D3 can promote both osteoclast activity, by influencing RANKL and NFATc1 signaling [ 57 ], and osteogenic activity, through BMP-2, Smad, Runx2, and the Wnt pathway [ 58 ].
In addition to its essential role in bone health, calcium, in the form of ionized calcium, is critical in a number of physiological functions, including neuronal function, muscle contraction, clotting, and intracellular signaling. Organisms cannot survive when such functions are compromised. As such, in conditions of low circulating calcium, bone undergoes increased resorption in order to supply circulating calcium ions for these life-sustaining functions. This is achieved predominantly via PTH [ 59 ], which stimulates bone resorption and increases the renal formation of active vitamin D to increase calcium absorption [ 60 ]. Conversely, calcitonin (CT) is a negative regulatory hormone of calcium. Secreted by thyroid C cells, CT inhibits the absorption of calcium from the intestine, promotes the excretion of calcium from the kidneys, and inhibits bone resorption, thereby reducing blood calcium levels [ 61 ].
Estrogen is critical for maintaining bone homeostasis. Its action is mediated primarily by the estrogen receptors ERα and ERβ, which are expressed in a variety of cells. Such receptors have been found to be widely expressed in osteocytes, osteoblasts, BM-MSCs, and osteoclasts. However, it is generally believed that estrogen’s bone-related activity occurs predominantly by influencing bone resorption to regulate bone remodeling [ 62 ]. Estrogen inhibits the secretion of RANKL and promotes the secretion of osteoclast-inhibiting factors such as growth hormone, GLP-1, and osteoprotegerin (OPG), thereby inhibiting osteoclast activity [ 11 ]. In addition to its primary role in inhibiting bone resorption, estrogen promotes osteogenic differentiation of MSCs and maintains the number of osteoblasts [ 11 ]. Therefore, estrogen deficiency, as in postmenopausal women, can lead to bone loss, which eventually progresses to osteoporosis. Hormone replacement therapy (HRT) has proven effective at preventing bone loss in postmenopausal women. In men [ 63 ], studies have found that testosterone can regulate bone metabolism directly and by being converted to estrogen [ 64 ]. Indeed, inhibition of aromatase, the enzyme responsible for androgen conversion into estrogen, resulted in decreased BMD in male rats [ 65 ].
Cytokines regulating bone metabolism
Cytokines provide another mechanism by which regulatory factors such as PTH and estrogen modulate bone remodeling, whereby such factors induce cells to release cytokines. A number of cytokines are involved in the regulation of bone metabolism, produced by bone cells themselves, as well as inflammatory cells and more. Osteoblasts secrete RANKL and OPG, as well as IL-1, IL-6, and TGF-β, to regulate the differentiation, activity, and apoptosis of osteoclasts [ 33 ]. Sclerotin, secreted by osteocytes, can prevent Wnt from binding to LRP5/LRP6, resulting in a decrease in β-catenin, thereby inhibiting bone formation [ 66 ]. Elsewhere, macrophages, endothelial cells, and fibroblasts secrete RANKL [ 67 ] and macrophage colony-stimulating factor (M-CSF) [ 68 ]. Knowledge of the cytokines that regulate bone metabolism has led to the development of novel osteoporosis treatments currently used in clinical settings, including RANKL monoclonal antibodies, sclerotin monoclonal antibodies, and cathepsin K inhibitors.
Emerging regulatory factors for bone remodeling
Our understanding of the factors that regulate bone remodeling is growing on a molecular, cellular, and whole organism level. In addition to the factors described above, increasing evidence has shown that noncoding RNAs [ 69 ], stem cells [ 14 ], bone marrow adipocytes [ 70 ], neuromodulation [ 71 ], exosomes [ 72 ], and gut microbiota [ 73 ] can also affect bone remodeling and participate in the process of bone metabolism. These factors may also underpin novel therapeutic avenues for osteoporosis, but their potential for translation into clinical applications is yet to be tested.
MicroRNAs (miRNAs) are noncoding, single-stranded RNA molecules encoded by endogenous genes to play a role in regulating posttranscriptional gene expression within bone cells. Namely, they regulate the expression of functional proteins in the bone activity signaling pathway [ 69 ]. Studies have found that other noncoding RNAs, such as long-noncoding RNAs (lncRNAs) and circular RNAs (circRNAs), are involved in the regulation of bone metabolism; however, miRNAs are the main subject of extensive and in-depth research [ 74 ]. For example, miRNA-21 (miR-21), which can be upregulated by RANKL, activates the PI3K/Akt signaling pathway by targeting PTEN [ 75 ] (a homologous gene of phosphate and tension on chromosome 10). This results in the promotion of osteoclastogenesis and bone resorption [ 76 ].
The relative activity and profile of miRNAs can differentially affect skeletal health and osteoporosis. Studies have shown that miR-31 [ 77 ], miR-103-3p [ 78 ], and miR-29b-3p [ 79 ] downregulate osteoblastic activity by inhibiting the expression of Runx2 [ 80 ]. Negative regulation of osteoblasts is also performed by miR-9-5p [ 81 ], miR-124 [ 82 ], and miR-203a-3p [ 83 ], which inhibit signal transduction of the Wnt signaling pathway, and miR-100 [ 84 ], which inhibits BMP signaling pathways. On the other hand, miR-194 [ 85 ], miR-874 [ 86 ], miR-96 [ 87 ], and miR-135-5p [ 88 ] can promote osteoblastic activity by stimulating Runx2, Wnt, and other molecules. Osteoclast activity is promoting by miR-21 [ 89 ], miR-183 [ 90 ], miR-155 [ 91 ], mir-148a [ 92 ], and miR-214 [ 93 ], which can inhibit the expression of RANKL, PI3K, TNF-α, and other molecules. miR-17 [ 94 ], miR-29 [ 95 ], and miR-503 [ 96 ] can downregulate osteoclast activity by inhibiting the RANKL signaling pathway. Elsewhere, miR-200a-3p [ 97 ], miR-449b-5p [ 98 ], and miR-579-3p [ 99 ] inhibit osteogenic differentiation of MSCs by affecting Runx2, chemokine receptor (CXCR), and other signaling pathway molecules. Studies investigating the wide-ranging effects of different miRNAs (Table 1 ), involving animal models and human cohort studies, have outlined their promise as a therapeutic target for osteoporosis. However, the translation to a clinical application is yet to be tested.
While the regulation of bone remodeling by miRNAs is a hot topic worthy of further clinical exploration, greater scientific knowledge is needed before entering the clinical application stage. Current miRNA research on osteoporosis is mostly limited to interventions at the cellular and animal levels. What is lacking is an in-depth exploration of the molecular interactions.
One such area of particular clinical interest for further study is the modulating effect of lncRNA and circRNA on miRNA. Both lncRNA and circRNA have miRNA-binding sites, which act as miRNA sponges in cells to counteract the inhibitory effect of miRNA on their target genes. Accordingly, this increases the expression level of target genes. This interaction can form a complex CeRNA (competing endogenous RNA) network, which plays an important role in various biological processes and disease progression. In osteoporosis, some lncRNAs and all circRNAs affect the differentiation of osteoblasts and osteoclasts by acting as miRNA sponges. The study of this interaction will help to analyze the pathogenesis of osteoporosis and the development of new drugs for the treatment of osteoporosis. For example, lncRNA TUR1 can further regulate osteoblast function by targeting PTEN as a synergistic effect of miR-21 [ 76 ]. circRNA-28313 can alleviate miR-195a by forming a CeRNA network to inhibit CSF1 (colony stimulating factor 1), functioning to regulate the osteoclast differentiation [ 13 ].
In addition to directly differentiating into osteoblasts, BM-MSCs can also act on osteoblasts and osteoclasts via a paracrine effect. Direct injection of stem cells in the treatment of osteoporosis is mainly found to operate via such paracrine mechanisms [ 106 ]. Stem cell therapy has been proven to be effective in animal model research. However, many problems remain to be solved to translate this treatment into clinical medicine, including stem cell extraction method, clinical ethics, allogeneic rejection, and so on. Stem cells have also been found to secrete exosomes as a means of intercellular regulation [ 72 ]. Exosomes are highly heterogeneous and contain a variety of proteins and RNAs. In light of their wide-ranging components and effects, their potential as an osteoporosis treatment requires further investigation.
Bone marrow adipocytes, bone endothelium, and bone nerves
In addition to bone itself, studies have also found that other tissues in bone, namely adipocytes, blood vessels, and nerves, can regulate bone remodeling. Bone marrow adipocytes may affect the development and function of other cell types in bone by secreting adipokines [ 70 ]. Some studies have reported that adipocyte conditioned medium samples inhibit the formation of osteogenic lineages of BM-MSCs and promote the formation of osteoclasts. Several key inhibitors of osteoblast differentiation have been identified as adipokines secreted by bone marrow adipocytes. Preadipocytes secrete curl-associated protein 1 (SFRP-1), which inhibits Wnt/β-catenin signals to reduce osteogenesis. Therefore, investigating ways to reduce the activity of bone marrow adipocytes and increase the proportion of bone marrow stem cells may hold promise as a new osteoporosis treatment [ 107 ]. Elsewhere, there is evidence that the growth of blood vessels in bone is coupled with osteogenesis [ 108 ]. Studies have found that bone endothelial cells secrete HIF-1α, which can affect bone angiogenesis and osteogenesis. Additionally, bone cells and bone endothelium and been observed to have a complementary interaction whereby osteoblasts release proangiogenic factors, which promotes angiogenesis and subsequently improves skeletal health [ 109 ]. SLIT3 was determined to be an osteoblast-derived angiogenic factor through transcriptome analysis [ 110 ]. In a postmenopausal osteoporosis mouse model, the use of recombinant SLIT3 can not only enhance fracture healing, but also offset bone loss. Other studies have found that nerve conduction signals in bone, such as cholinergic signals, may also be related to osteoporosis [ 111 ]. In osteoporotic rats, osteoblasts contained significantly decreased levels of muscarinic acetylcholine receptor (mACHR) M5 and M3. These findings provide evidence for the involvement of AChR signaling in osteoporosis [ 71 ]. This displays how intraosseous adipocytes, blood vessels, and nerves can all regulate bone metabolism and thus are implicated in the pathophysiology of osteoporosis. Although therapies based on this knowledge are not in the clinical stage, they may become a new treatment direction for the research and development of osteoporosis treatments.
Gut microbiota
Gut microbiota regulates human nutrition, metabolism, vitamin production, and immune system function, thus affecting bone metabolism [ 112 ]. Steroid hormones, PTH, and vitamin D metabolites may be affected by microbiota [ 73 ]. Additionally, compounds of bacterial origin, such as vitamins, may reach the blood and directly affect osteocyte activity. Further, the gut microbiota may affect host microRNAs (miRNAs) [ 113 ], such as miRNA-33-5p and miRNA-194, thereby influencing the development of osteoporosis [ 114 ]. Although this correlation between gut microbiota and bone metabolism has been found, whether bone physiology can be targeted through microbiota intervention requires further exploration. There are still many new targets being explored, such as platelet-derived growth factor-BB (PDGF-BB) [ 115 ] secreted by pre-osteoclasts, sphingosine-1-phosphate lyase [ 116 ], and integrin-β3 signaling [ 117 ], as some new research strategies are needed to enter clinical research.
Current research strategies
Current research strategies to find new target factors for osteoporosis treatment involve investigating the genomics, proteomics, epigenetics, and metabolomics of human samples [ 69 ]. To date, a large number of osteoporosis-associated genome-wide association studies (GWAS) have been carried out to identify the genetic risks of osteoporosis [ 118 ]. Several single-nucleotide polymorphisms (SNPs) have been revealed from the GWAS to be associated with low BMD and increased risks of osteoporotic fracture [ 119 ]. Mechanistically, these SNPs are believed to increase osteoporosis susceptibility via influencing the binding affinity of transcriptional factors or miRNAs [ 13 ]. For example, the genetic association between RANKL and BMD was reported through human GWAS [ 118 ]. This link was then explored at a cellular and whole organism level using animal models, and lastly assessed for clinical application. As a result, the link between RANKL and BMD gave rise to the RANKL-targeting drug denosumab, currently used in clinical settings for postmenopausal osteoporosis. This series of studies on the RNAKL–OPG system also highlights the importance of utilization of animal models in osteoporosis research that leads to the identification of new therapies. Several types of animals, including mice, rats, dogs, rabbits, and nonhuman primates, have been utilized in osteoporosis research [ 120 ]. Ovariectomized models (simulating postmenopausal osteoporosis), aging [ 121 ] and glucocorticoid-induced models (mimicking human glucocorticoid osteopenia) [ 122 ], and retinoic acid (RA)-induced models [ 120 ] are among the most widely used animal models. The knowledge gained from these animal models provides critical in vivo physiological and pathological evidence that reflects bone function and health in humans [ 123 ]. Importantly, the knowledge of the etiology, prevention, and treatment of osteoporosis obtained from these animal studies [ 124 ] could lead to the identification of new regulatory factors that could be developed as early diagnostic biomarkers and therapeutic targets for osteoporosis [ 125 ].
Pharmacologic strategies for osteoporosis
An understanding of the factors that regulate bone resorption and formation has allowed researchers to develop pharmacological agents to combat osteoporosis. Although there is a wide array of treatments available that have produced beneficial effects, many of these also come with disadvantages, as listed in Table 2 . This necessitates further research that both evaluates the value of current treatments and explores new therapeutic avenues that hopefully yield higher efficacy with fewer adverse effects.
Bone nutritional supplements: calcium and vitamin D
Adequate calcium intake is protective against osteoporosis and associated osteoporotic fractures. Calcium supplementation prevents the mobilization of bone calcium into the blood, reducing bone resorption and thus slowing bone loss [ 126 ]. Bone formation requires sufficient calcium to obtain an ideal bone peak, improve bone mineralization, and maintain bone health. Therefore, calcium supplements are a simple first-line treatment for osteoporosis. There is minimal risk of adverse effects, especially as the dosage should be adjusted according to the calcium intake of the population so as to prevent hypercalcemia [ 127 ]. However, calcium supplements alone cannot be used for the treatment of osteoporosis [ 128 ].
Vitamin D facilitates calcium absorption and can act directly on osteoblasts and osteoclasts to promote bone mineralization and inhibit bone resorption [ 129 ]. Studies have shown that vitamin D can prevent sarcopenia, improve muscle strength and postural stability, and reduce the risk of falls. Therefore, as with calcium, vitamin D is an essential nutrient for the treatment of osteoporosis, whose supplementary dose should be adjusted according to the vitamin D levels of the target population. Elderly individuals over 60 years of age need to increase their intake of vitamin D owing to a lack of sunshine and malabsorption of vitamin D. At present, vitamin D drugs include vitamin D, 25-hydroxy-vitamin D and 1,25 hydroxy-vitamin D. Hydroxy-vitamin D does not need to be activated by the liver and kidneys and can directly act on target organs. The effect of hydroxy-vitamin D is better than that of pure vitamin D, and it can also be taken by those with coexisting liver and/or kidney disease [ 130 ]. However, with this added benefit comes a higher financial cost. Similar to calcium supplements, the effect of vitamin D on osteoblasts and osteoclasts is not sufficient to treat osteoporosis on its own. Vitamin D needs to be used in combination with calcium and other anti-osteoporosis drugs. It is worth noting that excessive vitamin D intake can increase the blood calcium concentration above physiological levels. As a result, blood calcium can precipitate out as deposits in other organs and tissues, such as renal calcification, or even in the brain, causing deleterious effects [ 131 ].
Medications to inhibit bone resorption
Antiresorptive agents are currently the mainstay of osteoporosis treatment. They inhibit osteoclast activity by targeting a variety of processes involved in osteoclast function, thereby reducing bone resorption. These include bisphosphonates, estrogen, calcitonin, cathepsin K inhibitors, and RANKL inhibitors (Table 2 ).
Bisphosphonates
Bisphosphonates (BPs) are the first-line treatment for osteoporosis [ 132 ], taken in combination with calcium supplements [ 10 ]. BPs combine with hydroxyapatite on the bone surface, preventing cytokine release that would normally activate osteoclasts. As a result, osteoclasts cannot interact with the bone and they undergo increased apoptosis, causing reduced bone resorption [ 6 ]. This corresponds to effective clinical outcomes such as improved BMD and reduced rates of osteoporotic fractures [ 132 ]. Bisphosphonate drugs include both oral and intravenous forms. Given their proven results, many bisphosphonates are currently used in clinical settings: alendronate, zoledronate, risedronate, ibandronate, etidronate, and chlorophosphonate. However, studies have shown that long-term use of BPs may inhibit bone turnover and increase bone brittleness [ 133 ]. In addition, for patients with long-term use of BPs (usually > 3 years, with a median treatment time of 7 years), excessive inhibition of bone resorption can increase the risk of mandibular osteonecrosis or atypical femoral fracture [ 134 ].
Estrogen-related therapy
Estrogen replacement therapy (ET) and estrogen plus progesterone therapy (EPT) have been demonstrated to reduce bone loss and the risk of osteoporotic vertebral, nonvertebral, and medullary fractures in postmenopausal women [ 12 ]. Common estrogenic drugs are divided into natural and synthetic drugs. Natural estrogen drugs include estradiol, estriol, and estrone. Synthetic estrogen drugs include ethinylestradiol, ethinylether, and estradiol valerate, which have long-lasting effects. While estrogen replacement therapy is effective in reducing the risk of osteoporosis during menopause, long-term use of estrogen has been associated with increased risk of serious diseases [ 135 ] such as endometrial cancer, breast cancer, venous thrombosis, and stroke [ 136 ]. Combining this with progesterone, as in EPT, can alleviate some of these risks, particularly for endometrial cancers.
Selective estrogen receptor modulators (SERMs) provide another way of delivering the beneficial effects of estrogen replacement therapy while reducing estrogen-associated risks. SERMs bind to estrogen receptors in different tissues and, depending on the tissue type, can either produce agonistic or antagonistic biological effects [ 137 ]. For example, the SERM raloxifene has been found to play an agonistic role in bone tissue, where it inhibits bone resorption, increases bone density, and reduces the occurrence of vertebral fractures in postmenopausal women [ 138 ]. On the other hand, it has antagonistic effects on breast and uterine estrogen receptors [ 139 ]; by not stimulating breast or uterine tissue, it reduces the incidence of estrogen receptor-positive breast cancer and endometrial cancer [ 140 ]. This highlights a significant advantage of SERMs over traditional estrogen therapy. The use of SERMs in men has also been met with interest; however, it has so far been fraught with side effects and requires further exploration before clinical application [ 140 ].
Calcitonin for treatment
Calcitonin drugs used to treat osteoporosis include salcatonin and carbocalcitonin, which are extracted from salmon and eels. In addition to regulating calcium metabolism, calcitonin can also inhibit osteoclast proliferation and directly bind to them via calcitonin receptors to reduce osteoclast activity [ 61 ]. Administration of exogenous calcitonin inhibits bone resorption and improves BMD in patients with osteoporosis [ 141 ]. Furthermore, within the effective dose, combined with calcium and vitamin D supplementation, exogenous calcitonin does not reduce blood calcium levels. Within osteoporosis treatment, calcitonin has often been used more specifically to alleviate bone pain induced by osteoporosis. This benefit is activating endogenous opioid system and increasing β-endorphin concentration in the blood, providing analgesic effects. It can also inhibit the production of prostaglandins in local inflammatory tissues that act directly on the central nervous system pain receptors to produce analgesic effects [ 142 ].
Cathepsin K inhibitors
Cathepsin is a protease found in the cells (especially within lysosomes) of various animal tissues that hydrolyze proteins. Cathepsin K is a member of the cathepsin family and is expressed by osteoclasts, mainly functioning to degrade type I collagen in bone tissues [ 143 ]. It also promotes the inactivation and degradation of non-collagen factors, such as osteocalcin, osteopontin, osteonectin, proteoglycan, and related growth factors in bone tissue. The cathepsin K inhibitor odanacatib (ODN), developed by Merck (USA), inhibits this degradation of the bone matrix to treat osteoporosis [ 144 ]. Recent studies have found that ODN can increase the cortical thickness and bone mineral content of trabecular bone, thereby increasing BMD and bone load strength [ 145 ]. However, according to the long-term odanacatib fracture trial (LOFT), conducted at 388 centers across 40 countries involving over 16,000 participants [ 146 ], ODN was associated with significantly higher rates of atrial fibrillation and stroke. Owing to its unfavorable benefit–risk profile, it is rarely used clinically.
RANKL inhibitors
RANKL is one of the most important molecules involved in the regulation osteoclast activity. Denosumab, developed by Amgen (USA), is a fully human RANKL monoclonal antibody that prevents RANKL from activating its receptor on osteoclasts and pre-osteoclasts, leading to the inhibition of bone resorption and a subsequent increase in bone mass. Compared with BPs, denosumab can improve BMD more quickly, including in cortical and cancellous bone, and reduce the risk of fracture [ 7 ]. Clinical studies have found that an increase in bone density can still be observed after 10 years of denosumab treatment, which is better than that observed with BP drugs. However, studies have shown that denosumab discontinuation causes a rapid decline in BMD due to a rebound activity in osteoclasts, leading to an increase in the incidence of multiple vertebral fractures [ 8 ]. This phenomenon is called “drug holiday” [ 147 ]. It is suggested that denosumab should be used continuously if it is tolerated, and in the event of discontinuation, a stepwise approach or combination with other therapies such as bone-forming drugs should be considered to reduce or prevent rebound bone loss and fracture [ 147 ]. Similar to using bisphosphonates, long-term use with denosumab will still increase the risk of mandibular osteonecrosis and atypical femoral fractures, owing to excessive inhibition of bone resorption [ 148 ].
Drugs that promote bone formation
Compared with antiresorptive drugs, there are fewer osteoporosis medications on the market that work by promoting bone formation. However, such drugs that target osteoblasts and operate via anabolic actions are described below and summarized in Table 2 .
Parathyroid hormone (PTH) analogues
PTH promotes bone resorption when blood calcium levels decrease [ 59 ]. However, intermittent low-dose use of PTH analogues (PTHA) has been shown to stimulate osteoblast activity and promote osteogenic activity [ 149 ]. As the dose increases, it can also stimulate osteoclast activity, inducing bone resorption instead [ 150 ]. Teriparatide is an active fragment of recombinant human PTH 1–34 (rhPTH1–34) [ 151 ]. Treating osteoporosis with teriparatide alone causes the bone metabolic rate to increase significantly in the first 6 months. This corresponds with an increase in bone mass, especially cortical bone resorption holes, but also a transient decrease in bone strength, especially in the hip bone [ 151 ]. As such, PTHAs are suitable for patients with vertebral fractures or extremely low bone density, where PTHAs can quickly increase bone density, but they must be combined with BPs to maintain bone density long term [ 152 ].
Anti-sclerotin antibody
Sclerotin is secreted by osteocytes and inhibits bone formation by inhibiting the Wnt signaling pathway y[ 153 ]. Romosozumab is a monoclonal antibody against sclerotin, which was developed by Amgen (USA) and approved by the US Food and Drug Administration (FDA) in 2019 [ 66 ]. It improves osteoporosis by reducing sclerotin expression or inhibiting its effect on the Wnt signaling pathway in osteoblasts [ 66 ]. In some countries, including Japan and Germany, it has now entered clinical applications for the treatment of osteoporosis in postmenopausal women with a high fracture risk [ 154 ]. In a phase III trial, compared with placebo and oral alendronate, the use of romosozumab for 12 months significantly reduced the risk of vertebral body and clinical fractures in postmenopausal women with osteoporosis. After further follow-up for 12–24 months, the risk of fracture also improved significantly [ 155 ]. However, owing to the short clinical application time, there are insufficient clinical data to fully evaluate the efficacy and side effects of this drug.
Drug targets with bidirectional regulation
Strontium is a trace element in the human body, almost entirely located in bone [ 156 ]. Strontium exerts an anti-osteoporotic effect by promoting osteoblasts, inhibiting osteoclasts, and regulating MSCs[ 157 ]. Strontium ranelate (SrR) is a strontium salt drug used clinically [ 158 ], proven to be more effective in treating postmenopausal osteoporosis than 25-hydroxy-vitamin D [ 156 ]. However, SrR can cause a number of adverse reactions, including skin damage, ischemic heart disease, peripheral vascular disease, and cerebrovascular disease [ 159 ]. This is a major reason why SrR is not widely used in the treatment of osteoporosis [ 160 ].
Potential novel therapeutic targets for osteoporosis
Despite having a variety of drugs available on the market, current pharmacological treatments for osteoporosis are either relatively ineffective or unsafe. Therefore, new treatments that produce better clinical outcomes with fewer adverse effects are urgently needed. In recent years, treatments based on stem cells and miRNA, as well as bone-targeting methods, have received increasing interest as novel therapeutic avenues for osteoporosis.
- Stem cell therapy
Stem cell therapy is an emerging new treatment approach that harnesses stem cells’ great potential to differentiate and regulate intercellular communication. The stem cells used for research can come from different sources, including embryonic stem cells (ESCs), adult stem cells (ASCs), and induced pluripotent stem cells (iPSCs) [ 161 ]. So far, stem cell therapy has provided a great opportunity for degenerative disease and diseases that require tissue regeneration, such as stroke, premature ovarian failure, and spinal cord injury [ 162 ]. The main aim of stem cell therapy in osteoporosis treatment is to promote bone formation, rather than reducing resorption [ 163 ]. This is currently being explored via many in vivo animal studies as summarized in Table 3 . Stem cells have the ability to differentiate into osteoblasts, promote the growth of osteoblasts, and inhibit the activity of osteoclasts through cell-to-cell interactions, using cytokines, chemokines, and extracellular vesicles [ 14 ]. From these actions, stem cells can reverse degenerative damage to bone by improving cell lifespan and activity. Currently, this therapy is still in the early stages of cell and animal experimentation. In rat and rabbit models, injection of stem cells can improve the microstructure of osteoporotic bone tissue, increase bone density, and increase the osteogenic activity of alkaline phosphatase (ALP) and osteocalcin (OCN) [ 164 ]. Additionally, the injection of stem cells can also promote the expression of OPG and inhibit TNF-α and RANKL, demonstrating improved osteogenic differentiation ability [ 165 ]. Owing to ethical issues, it is difficult to conduct experiments with ESCs and iPSCs in human research. Adult stem cells such as BM-MSCs, adipose stem cells (ADSCs), and hematopoietic stem cells do not involve such ethical barriers, and are also highly available [ 166 ] and amenable to clinical transformation [ 167 ]. However, their differentiation potential is generally weaker than that of ESCs, and the problem of stem cell homing after injection remains unsolved. At present, in animal models, the chemotactic ability of implanted stem cells can be improved by overexpression of chemokines such as CXCR4 or RANK-Fc [ 168 ]. Another shortcoming of stem cell treatment is the unwanted differentiation of transplanted MSCs and their potential to suppress antitumor immune responses, in addition to generating new blood vessels that may promote tumor growth and metastasis [ 162 ]. According to previous reports [ 169 ], stem cells have been used to treat human patients with osteogenesis imperfecta. To further develop stem cell therapy as a bona fide clinical treatment for osteoporosis, it will be necessary to increase its safety, especially in relation to their oncogenic effects [ 162 ].
miRNA-based therapy
miRNA-based therapy has shown potential in the treatment of osteoporosis and osteoporotic fracture [ 176 ]. There are abundant in vitro studies in which miRNA mimetics or inhibitors have been used to treat MSCs, osteoblasts, or osteoclasts [ 177 ] to determine the relationships between miRNAs and bone cell activity [ 178 ]. However, few in vivo studies have been conducted. Current in vivo experiments have mostly used miRNA inhibitors, lentiviral transfection, or exosomes to intervene in rat or mouse osteoporosis models. Following this, the bone quality of the rodents was observed to determine whether the regulation of miRNA influenced osteoporotic progression [ 87 ]. The clinical translation of lentivirus transfection can be difficult, but the clinical transformation of inhibitors is possible. Currently, the most widely used miRNA inhibitors are modified nucleoside oligomers, such as anti-miRNA oligonucleotides (AMOs) [ 179 ], locked nuclear acid (LNA) AMOs, antagomirs [ 180 ], and miRNA sponges [ 181 ]. The binding of miRNAs and target mRNAs is competitively inhibited using the principle of complementarity with the target miRNA sequence. However, the exploration of chemical small-molecule inhibitors for miRNA is still in its infancy, and few are used in osteoporosis models. For example, studies have shown that, compared with the ovariectomized (OVX) group, the BMD of rats treated with miR-30a-3p inhibitors was significantly increased; the miR-30a-3p inhibitor significantly upregulated bone volume/total volume (BV/TV), trabecular number (TB. N), and trabecular thickness (TB. Th) in OVX rats [ 182 ]. Since miRNAs can affect multiple signaling pathways, this could result in off-target activity and corresponding adverse effects. Therefore, further research is needed to develop tissue-specific miRNA inhibitors for osteoporosis treatment.
Bone-specific targeting technology
Regardless of the drug class, the ability to target bone specifically remains a highly significant barrier to overcome in order to advance osteoporosis therapy [ 183 ]. Owing to their ability to specifically bind with hydroxyapatite, bisphosphonates have been engineered for combination drug use, whereby they act like a vehicle to help other agents target bone tissue. Previous studies have combined iron oxide nanoparticles with bisphosphonates to deliver them to bone tissue. The iron oxide nanoparticles then exert their anti-osteoporotic action by removing active oxygen in bone to promote osteogenesis, which also occurs synergistically with bisphosphonates’ antiresorptive effect [ 184 ]. Elsewhere, exosomes have shown promise in providing bone-specific targeting. Exosomes secreted by BM-MSC have been engineered to both contain siSHN3 and modify the bone-targeting peptide. This enables them to specifically combine with osteoblasts to promote the expression of SLIT3 (vascular endothelial growth factor). As mentioned earlier, SLIT3 can not only enhance fracture healing, but also offset bone loss to treat osteoporosis [ 185 ]. In stem cell therapy, the overexpression of chemokine CXCR4 in transplanted MSCs has also been shown to improve the stem cell tracking to bone [ 168 ]. This displays how engineering bone-targeting technologies is an important branch of osteoporosis research, with the potential to produce highly specific and effective treatments.
Conclusions and outlook
Bone remodeling requires a finely tuned balance of bone resorption and formation to maintain bone health. The control of this process involves an orchestrated web of regulation at a molecular and cellular level. When the bone remodeling balance is skewed toward increased resorption, this leads to osteoporosis. Such mechanisms underlying bone remodeling are explored through new research technologies such as genomics and proteomics. Deepening the understanding of the molecular mechanisms of bone remodeling has led to the development of various osteoporosis therapeutics that promote bone formation, inhibit bone resorption, or both.
In the treatment of osteoporosis, bisphosphonates combined with bone nutrients are the first-line treatments. The discovery of RANKL monoclonal antibodies and other novel drugs in the market shows how research into osteoporosis drug development is a fruitful area with further therapeutic potential. With the advancement of molecular biology and pharmacology, safer and more effective osteoporosis treatment options will continue to be identified and developed. We expect that clinical translational research employing new therapeutic methods such as stem cell therapy, miRNA inhibitors, and bone-targeting technology will bring breakthroughs in the treatment of osteoporosis.
Availability of data and materials
Not applicable.
Abbreviations
American Association of Clinical Endocrinologists
Adipose-derived mesenchymal stem cells
Protein kinase B
Anti-miRNA oligonucleotides
Activator protein-1
Bone mineral density
Bone morphogenetic proteins
Bone volume/total volume
Core binding factor α 1
Chemokine receptor
Extracellular regulated protein kinases
Embryonic stem cells
Green fluorescent protein
Interleukin-1
Induced pluripotent stem cells
NF-κB inhibitor-κ-binding kinases
c-Jun N-terminal kinase
Leucine-rich G-protein-coupled receptor 4
Low-density lipoprotein receptor-related protein
Mucosa-associated lymphoid tissue lymphoma translocation factor 1
Mitogen-activated protein kinases
Macrophage colony-stimulating factor
Matrix metalloproteinase
Mesenchymal stem cells
Nuclear factor of activated T cells 1
NF-κB inducible kinase
Nonobese diabetic/severe combined immunodeficient
Osteoprotegerin
Osteopontin
Ovariectomized
Phosphatidylinositol 3-kinase
Polylactic acid polyglycolic acid copolymer/collagen type I
Protein phosphatase 2A
Gene of phosphate and tension homology deleted on chromosome 10
Parathyroid hormone
Nuclear factor kappa B (NF-κB) ligand activated receptor
Transcription factor runt-related transcription factor 2
Selective estrogen receptor modulators
Trabecular number
Trabecular thickness
Transforming growth factor-β
Tumor necrosis factor-α
Tumor necrosis factor receptor-associated factor-6
Umbilical cord blood
Very small embryonic-like cells
Sietsema DL. Fighting the epidemic: bone health and osteoporosis. Nurs Clin North Am. 2020;55(2):193–202.
Article PubMed Google Scholar
NIH Consensus Development Panel on Osteoporosis Prevention, Diagnosis, and Therapy, March 7-29, 2000: highlights of the conference. South Med J. 2001;94(6):569–73.
Osnes EK, Lofthus CM, Meyer HE, Falch JA, Nordsletten L, Cappelen I, et al. Consequences of hip fracture on activities of daily life and residential needs. Osteoporos Int. 2004;15(7):567–74.
Article CAS PubMed Google Scholar
Kanis JA, Harvey NC, Cooper C, Johansson H, Odén A, McCloskey EV. A systematic review of intervention thresholds based on FRAX: a report prepared for the National Osteoporosis Guideline Group and the International Osteoporosis Foundation. Arch Osteoporos. 2016;11(1):25.
Article PubMed PubMed Central Google Scholar
Kenkre JS, Bassett J. The bone remodelling cycle. Ann Clin Biochem. 2018;55(3):308–27.
Cremers S, Drake MT, Ebetino FH, Bilezikian JP, Russell RGG. Pharmacology of bisphosphonates. Br J Clin Pharmacol. 2019;85(6):1052–62.
Deeks ED. Denosumab: a review in postmenopausal osteoporosis. Drugs Aging. 2018;35(2):163–73.
Bone HG, Wagman RB, Brandi ML, Brown JP, Chapurlat R, Cummings SR, et al. 10 years of denosumab treatment in postmenopausal women with osteoporosis: results from the phase 3 randomised FREEDOM trial and open-label extension. Lancet Diabetes Endocrinol. 2017;5(7):513–23.
Anagnostis P, Paschou SA, Mintziori G, Ceausu I, Depypere H, Lambrinoudaki I, et al. Drug holidays from bisphosphonates and denosumab in postmenopausal osteoporosis: EMAS position statement. Maturitas. 2017;101:23–30.
Camacho PM, Petak SM, Binkley N, Diab DL, Eldeiry LS, Farooki A, et al. American Association of Clinical Endocrinologists/American College of Endocrinology Clinical practice guidelines for the diagnosis and treatment of postmenopausal osteoporosis-2020 update. Endocr Pract. 2020;26(Suppl 1):1–46.
Li L, Wang Z. Ovarian aging and osteoporosis. Adv Exp Med Biol. 2018;1086:199–215.
Levin VA, Jiang X, Kagan R. Estrogen therapy for osteoporosis in the modern era. Osteoporos Int. 2018;29(5):1049–55.
Yang Y, Yujiao W, Fang W, Linhui Y, Ziqi G, Zhichen W, et al. The roles of miRNA, lncRNA and circRNA in the development of osteoporosis. Biol Res. 2020;53(1):40.
Article CAS PubMed PubMed Central Google Scholar
Paspaliaris V, Kolios G. Stem cells in osteoporosis: from biology to new therapeutic approaches. Stem Cells Int. 2019;2019:1730978.
Article PubMed PubMed Central CAS Google Scholar
Li F, Zhou C, Xu L, Tao S, Zhao J, Gu Q. Effect of stem cell therapy on bone mineral density: a meta-analysis of preclinical studies in animal models of osteoporosis. PLoS ONE. 2016;11(2): e0149400.
Adamopoulos IE. Inflammation in bone physiology and pathology. Curr Opin Rheumatol. 2018;30(1):59–64.
Weitzmann MN. Bone and the immune system. Toxicol Pathol. 2017;45(7):911–24.
Ramchand SK, Seeman E. Reduced bone modeling and unbalanced bone remodeling: targets for antiresorptive and anabolic therapy. Handb Exp Pharmacol. 2020;262:423–50.
Compston JE, McClung MR, Leslie WD. Osteoporosis. Lancet. 2019;393(10169):364–76.
Ono T, Nakashima T. Recent advances in osteoclast biology. Histochem Cell Biol. 2018;149(4):325–41.
Lerner UH, Kindstedt E, Lundberg P. The critical interplay between bone resorbing and bone forming cells. J Clin Periodontol. 2019;46(Suppl 21):33–51.
Tobeiha M, Moghadasian MH, Amin N, Jafarnejad S. RANKL/RANK/OPG pathway: a mechanism involved in exercise-induced bone remodeling. Biomed Res Int. 2020;2020:6910312.
McDonald MM, Khoo WH, Ng PY, Xiao Y, Zamerli J, Thatcher P, et al. Osteoclasts recycle via osteomorphs during RANKL-stimulated bone resorption. Cell. 2021;184(5):1330-47.e13.
Qin S, Zhang Q, Zhang L. Effect of OPG gene mutation on protein expression and biological activity in osteoporosis. Exp Ther Med. 2017;14(2):1475–80.
Liao HJ, Tsai HF, Wu CS, Chyuan IT, Hsu PN. TRAIL inhibits RANK signaling and suppresses osteoclast activation via inhibiting lipid raft assembly and TRAF6 recruitment. Cell Death Dis. 2019;10(2):77.
Zhou L, Song H, Zhang Y, Ren Z, Li M, Fu Q. Polyphyllin VII attenuated RANKL-induced osteoclast differentiation via inhibiting of TRAF6/c-Src/PI3K pathway and ROS production. BMC Musculoskelet Disord. 2020;21(1):112.
Zhi X, Fang C, Gu Y, Chen H, Chen X, Cui J, et al. Guaiacol suppresses osteoclastogenesis by blocking interactions of RANK with TRAF6 and C-Src and inhibiting NF-kappaB, MAPK and AKT pathways. J Cell Mol Med. 2020;24(9):5122–34.
Cui J, Li X, Wang S, Su Y, Chen X, Cao L, et al. Triptolide prevents bone loss via suppressing osteoclastogenesis through inhibiting PI3K–AKT–NFATc1 pathway. J Cell Mol Med. 2020;24(11):6149–61.
Anesi A, Generali L, Sandoni L, Pozzi S, Grande A. From osteoclast differentiation to osteonecrosis of the jaw: molecular and clinical insights. Int J Mol Sci. 2019;20(19):4925.
Article CAS PubMed Central Google Scholar
Wang L, Bai J, Wang Q, Ge G, Lin J, Xu N, et al. Inhibition of protein phosphatase 2A attenuates titanium-particle induced suppression of bone formation. Int J Biol Macromol. 2020;142:142–51.
Jang Y, Sohn HM, Ko YJ, Hyun H, Lim W. Inhibition of RANKL-induced osteoclastogenesis by novel mutant RANKL. Int J Mol Sci. 2021;22(1):434.
Wang T, He C. TNF-alpha and IL-6: the link between immune and bone system. Curr Drug Targets. 2020;21(3):213–27.
CAS PubMed Google Scholar
Amarasekara DS, Yun H, Kim S, Lee N, Kim H, Rho J. Regulation of osteoclast differentiation by cytokine networks. Immune Netw. 2018;18(1): e8.
Marahleh A, Kitaura H, Ohori F, Kishikawa A, Ogawa S, Shen WR, et al. TNF-alpha directly enhances osteocyte RANKL expression and promotes osteoclast formation. Front Immunol. 2019;10:1–12.
Article CAS Google Scholar
Meloni L, Verstrepen L, Kreike M, Staal J, Driege Y, Afonina IS, et al. Mepazine inhibits RANK-induced osteoclastogenesis independent of its MALT1 inhibitory function. Molecules. 2018;23(12):3144.
Article PubMed Central CAS Google Scholar
Monajemi M, Fisk S, Pang YCF, Leung J, Menzies SC, Ben-Othman R, et al. Malt1 deficient mice develop osteoporosis independent of osteoclast-intrinsic effects of Malt1 deficiency. J Leukoc Biol. 2019;106(4):863–77.
Hu L, Yin C, Zhao F, Ali A, Ma J, Qian A. Mesenchymal stem cells: cell fate decision to osteoblast or adipocyte and application in osteoporosis treatment. Int J Mol Sci. 2018;19(2):360.
Blair HC, Larrouture QC, Li Y, Lin H, Beer-Stoltz D, Liu L, et al. Osteoblast differentiation and bone matrix formation in vivo and in vitro. Tissue Eng Part B Rev. 2017;23(3):268–80.
Agostino M, Pohl S. The structural biology of canonical Wnt signalling. Biochem Soc Trans. 2020;48(4):1765–80.
Du JH, Lin SX, Wu XL, Yang SM, Cao LY, Zheng A, et al. The function of Wnt ligands on osteocyte and bone remodeling. J Dent Res. 2019;98(8):930–8.
Sawakami K, Robling AG, Ai M, Pitner ND, Liu D, Warden SJ, et al. The Wnt co-receptor LRP5 is essential for skeletal mechanotransduction but not for the anabolic bone response to parathyroid hormone treatment. J Biol Chem. 2006;281(33):23698–711.
Karner CM, Long F. Wnt signaling and cellular metabolism in osteoblasts. Cell Mol Life Sci. 2017;74(9):1649–57.
Moorer MC, Riddle RC. Regulation of osteoblast metabolism by Wnt signaling. Endocrinol Metab (Seoul). 2018;33(3):318–30.
Lowery JW, Rosen V. The BMP pathway and its inhibitors in the skeleton. Physiol Rev. 2018;98(4):2431–52.
Zou ML, Chen ZH, Teng YY, Liu SY, Jia Y, Zhang KW, et al. The Smad dependent TGF-beta and BMP signaling pathway in bone remodeling and therapies. Front Mol Biosci. 2021;8:1–11.
Google Scholar
Garcia J, Delany AM. MicroRNAs regulating TGFbeta and BMP signaling in the osteoblast lineage. Bone. 2021;143(115791):1–8.
Bal Z, Kushioka J, Kodama J, Kaito T, Yoshikawa H, Korkusuz P, et al. BMP and TGFbeta use and release in bone regeneration. Turk J Med Sci. 2020;50:1707–22.
Onodera S, Saito A, Hojo H, Nakamura T, Zujur D, Watanabe K, et al. Hedgehog activation regulates human osteoblastogenesis. Stem Cell Rep. 2020;15(1):125–39.
Qin X, Jiang Q, Miyazaki T, Komori T. Runx2 regulates cranial suture closure by inducing hedgehog, Fgf, Wnt and Pthlh signaling pathway gene expressions in suture mesenchymal cells. Hum Mol Genet. 2019;28(6):896–911.
Komori T. Molecular mechanism of Runx2-dependent bone development. Mol Cells. 2020;43(2):168–75.
CAS PubMed PubMed Central Google Scholar
Tu X, Joeng KS, Long F. Indian hedgehog requires additional effectors besides Runx2 to induce osteoblast differentiation. Dev Biol. 2012;362(1):76–82.
Xu Y, Shu B, Tian Y, Chelly M, Morandi MM, Barton S, et al. Notch activation promotes osteoblast mineralization by inhibition of apoptosis. J Cell Physiol. 2018;233(10):6921–8.
Yu J, Canalis E. Notch and the regulation of osteoclast differentiation and function. Bone. 2020;138: 115474.
Urbanek K, Lesiak M, Krakowian D, Koryciak-Komarska H, Likus W, Czekaj P, et al. Notch signaling pathway and gene expression profiles during early in vitro differentiation of liver-derived mesenchymal stromal cells to osteoblasts. Lab Invest. 2017;97(10):1225–34.
Christakos S, Li S, DeLa CJ, Verlinden L, Carmeliet G. Vitamin D and bone. Handb Exp Pharmacol. 2020;262:47–63.
Shymanskyi I, Lisakovska O, Mazanova A, Labudzynskyi D, Veliky M. Vitamin D3 modulates impaired crosstalk between RANK and glucocorticoid receptor signaling in bone marrow cells after chronic prednisolone administration. Front Endocrinol (Lausanne). 2018;9:1–14.
Article Google Scholar
Zarei A, Morovat A, Javaid K, Brown CP. Vitamin D receptor expression in human bone tissue and dose-dependent activation in resorbing osteoclasts. Bone Res. 2016;4:16030.
Shi YC, Worton L, Esteban L, Baldock P, Fong C, Eisman JA, et al. Effects of continuous activation of vitamin D and Wnt response pathways on osteoblastic proliferation and differentiation. Bone. 2007;41(1):87–96.
Wein MN, Kronenberg HM. Regulation of bone remodeling by parathyroid hormone. Cold Spring Harb Perspect Med. 2018;8(8):a031237.
Goltzman D, Mannstadt M, Marcocci C. Physiology of the calcium–parathyroid hormone–vitamin D axis. Front Horm Res. 2018;50:1–13.
Naot D, Musson DS, Cornish J. The activity of peptides of the calcitonin family in bone. Physiol Rev. 2019;99(1):781–805.
Khosla S, Oursler MJ, Monroe DG. Estrogen and the skeleton. Trends Endocrinol Metab. 2012;23(11):576–81.
Cauley JA. Estrogen and bone health in men and women. Steroids. 2015;99(Pt A):11–5.
Vilaca T, Eastell R, Schini M. Osteoporosis in men. Lancet Diabetes Endocrinol. 2022;10(4):273–83.
Matsumoto C, Inada M, Toda K, Miyaura C. Estrogen and androgen play distinct roles in bone turnover in male mice before and after reaching sexual maturity. Bone. 2006;38(2):220–6.
Bandeira L, Lewiecki EM, Bilezikian JP. Romosozumab for the treatment of osteoporosis. Expert Opin Biol Ther. 2017;17(2):255–63.
Hu Y, Li X, Zhi X, Cong W, Huang B, Chen H, et al. RANKL from bone marrow adipose lineage cells promotes osteoclast formation and bone loss. EMBO Rep. 2021;22(7): e52481.
Chen X, Zhi X, Wang J, Su J. RANKL signaling in bone marrow mesenchymal stem cells negatively regulates osteoblastic bone formation. Bone Res. 2018;6:34.
Yang TL, Shen H, Liu A, Dong SS, Zhang L, Deng FY, et al. A road map for understanding molecular and genetic determinants of osteoporosis. Nat Rev Endocrinol. 2020;16(2):91–103.
Muruganandan S, Govindarajan R, Sinal CJ. Bone marrow adipose tissue and skeletal health. Curr Osteoporos Rep. 2018;16(4):434–42.
Wan QQ, Qin WP, Ma YX, Shen MJ, Li J, Zhang ZB, et al. Crosstalk between bone and nerves within bone. Adv Sci (Weinh). 2021;8(7):2003390.
He XY, Yu HM, Lin S, Li YZ. Advances in the application of mesenchymal stem cells, exosomes, biomimetic materials, and 3D printing in osteoporosis treatment. Cell Mol Biol Lett. 2021;26(1):47.
D’Amelio P, Sassi F. Gut microbiota, immune system, and bone. Calcif Tissue Int. 2018;102(4):415–25.
Chen YS, Lian WS, Kuo CW, Ke HJ, Wang SY, Kuo PC, et al. Epigenetic regulation of skeletal tissue integrity and osteoporosis development. Int J Mol Sci. 2020;21(14):4923.
Li FP, Lin DQ, Gao LY. LncRNA TUG1 promotes proliferation of vascular smooth muscle cell and atherosclerosis through regulating miRNA-21/PTEN axis. Eur Rev Med Pharmacol Sci. 2018;22(21):7439–47.
PubMed Google Scholar
Han Y, Liu C, Lei M, Sun S, Zheng W, Niu Y, et al. LncRNA TUG1 was upregulated in osteoporosis and regulates the proliferation and apoptosis of osteoclasts. J Orthop Surg Res. 2019;14(1):416.
Mäkitie RE, Hackl M, Niinimäki R, Kakko S, Grillari J, Mäkitie O. Altered microRNA profile in osteoporosis caused by impaired WNT signaling. J Clin Endocrinol Metab. 2018;103(5):1985–96.
Kocijan R, Weigl M, Skalicky S, Geiger E, Ferguson J, Leinfellner G, et al. MicroRNA levels in bone and blood change during bisphosphonate and teriparatide therapy in an animal model of postmenopausal osteoporosis. Bone. 2020;131: 115104.
Zeng Q, Wang Y, Gao J, Yan Z, Li Z, Zou X, et al. miR-29b-3p regulated osteoblast differentiation via regulating IGF-1 secretion of mechanically stimulated osteocytes. Cell Mol Biol Lett. 2019;24:11.
Bottani M, Banfi G, Lombardi G. Perspectives on miRNAs as epigenetic markers in osteoporosis and bone fracture risk: a step forward in personalized diagnosis. Front Genet. 2019;10:1044.
Zhang HG, Wang XB, Zhao H, Zhou CN. MicroRNA-9-5p promotes osteoporosis development through inhibiting osteogenesis and promoting adipogenesis via targeting Wnt3a. Eur Rev Med Pharmacol Sci. 2019;23:456–63.
Qadir AS, Um S, Lee H, Baek K, Seo BM, Lee G, et al. miR-124 negatively regulates osteogenic differentiation and in vivo bone formation of mesenchymal stem cells. J Cell Biochem. 2015;116(5):730–42.
Fan FY, Deng R, Qiu L, Wen Q, Zeng Y, Gao L, et al. miR-203a-3p.1 is involved in the regulation of osteogenic differentiation by directly targeting Smad9 in MM-MSCs. Oncol Lett. 2019;18(6):6339–46.
Fu HL, Pan HX, Zhao B, Dong BC, Shao L, Fu GS, et al. MicroRNA-100 inhibits bone morphogenetic protein-induced osteoblast differentiation by targeting Smad1. Eur Rev Med Pharmacol Sci. 2016;20(18):3911–9.
Li J, He X, Wei W, Zhou X. MicroRNA-194 promotes osteoblast differentiation via downregulating STAT1. Biochem Biophys Res Commun. 2015;460(2):482–8.
Lin JC, Liu ZG, Yu B, Zhang XR. MicroRNA-874 targeting SUFU involves in osteoblast proliferation and differentiation in osteoporosis rats through the Hedgehog signaling pathway. Biochem Biophys Res Commun. 2018;506(1):194–203.
Ma S, Wang DD, Ma CY, Zhang YD. MicroRNA-96 promotes osteoblast differentiation and bone formation in ankylosing spondylitis mice through activating the Wnt signaling pathway by binding to SOST. J Cell Biochem. 2019;120(9):15429–42.
Yin N, Zhu L, Ding L, Yuan J, Du L, Pan M, et al. miR-135-5p promotes osteoblast differentiation by targeting HIF1AN in MC3T3-E1 cells. Cell Mol Biol Lett. 2019;24:51.
Wang S, Liu Z, Wang J, Ji X, Yao Z, Wang X. miR21 promotes osteoclastogenesis through activation of PI3K/Akt signaling by targeting Pten in RAW264.7 cells. Mol Med Rep. 2020;21(3):1125–32.
Ke K, Sul OJ, Rajasekaran M, Choi HS. MicroRNA-183 increases osteoclastogenesis by repressing heme oxygenase-1. Bone. 2015;81:237–46.
Mao Z, Zhu Y, Hao W, Chu C, Su H. MicroRNA-155 inhibition up-regulates LEPR to inhibit osteoclast activation and bone resorption via activation of AMPK in alendronate-treated osteoporotic mice. IUBMB Life. 2019;71(12):1916–28.
Xiao Y, Li B, Liu J. MicroRNA148a inhibition protects against ovariectomy-induced osteoporosis through PI3K/AKT signaling by estrogen receptor alpha. Mol Med Rep. 2018;17(6):7789–96.
Sun Y, Kuek V, Liu Y, Tickner J, Yuan Y, Chen L, et al. miR-214 is an important regulator of the musculoskeletal metabolism and disease. J Cell Physiol. 2018;234(1):231–45.
Article PubMed CAS Google Scholar
Shi C, Qi J, Huang P, Jiang M, Zhou Q, Zhou H, et al. MicroRNA-17/20a inhibits glucocorticoid-induced osteoclast differentiation and function through targeting RANKL expression in osteoblast cells. Bone. 2014;68:67–75.
Lian WS, Ko JY, Chen YS, Ke HJ, Hsieh CK, Kuo CW, et al. MicroRNA-29a represses osteoclast formation and protects against osteoporosis by regulating PCAF-mediated RANKL and CXCL12. Cell Death Dis. 2019;10(10):705.
Chen C, Cheng P, Xie H, Zhou HD, Wu XP, Liao EY, et al. miR-503 regulates osteoclastogenesis via targeting RANK. J Bone Miner Res. 2014;29(2):338–47.
Lv R, Pan X, Song L, Sun Q, Guo C, Zou S, et al. MicroRNA-200a-3p accelerates the progression of osteoporosis by targeting glutaminase to inhibit osteogenic differentiation of bone marrow mesenchymal stem cells. Biomed Pharmacother. 2019;116: 108960.
Li JY, Wei X, Sun Q, Zhao XQ, Zheng CY, Bai CX, et al. MicroRNA-449b-5p promotes the progression of osteoporosis by inhibiting osteogenic differentiation of BMSCs via targeting Satb2. Eur Rev Med Pharmacol Sci. 2019;23(15):6394–403.
Luo B, Yang JF, Wang YH, Qu GB, Hao PD, Zeng ZJ, et al. MicroRNA-579-3p promotes the progression of osteoporosis by inhibiting osteogenic differentiation of mesenchymal stem cells through regulating Sirt1. Eur Rev Med Pharmacol Sci. 2019;23(16):6791–9.
Shi X, Zhang Z. MicroRNA-135a-5p is involved in osteoporosis progression through regulation of osteogenic differentiation by targeting RUNX2. Exp Ther Med. 2019;18(4):2393–400.
Du F, Wu H, Zhou Z, Liu YU. MicroRNA-375 inhibits osteogenic differentiation by targeting runt-related transcription factor 2. Exp Ther Med. 2015;10(1):207–12.
Kureel J, Dixit M, Tyagi AM, Mansoori MN, Srivastava K, Raghuvanshi A, et al. miR-542-3p suppresses osteoblast cell proliferation and differentiation, targets BMP-7 signaling and inhibits bone formation. Cell Death Dis. 2014;5(2): e1050.
Li X, Ning L, Zhao X, Wan S. MicroRNA-543 promotes ovariectomy-induced osteoporosis through inhibition of AKT/p38 MAPK signaling pathway by targeting YAF2. J Cell Biochem. 2018;120:8561.
Li H, Li T, Fan J, Li T, Fan L, Wang S, et al. miR-216a rescues dexamethasone suppression of osteogenesis, promotes osteoblast differentiation and enhances bone formation, by regulating c-Cbl-mediated PI3K/AKT pathway. Cell Death Differ. 2015;22(12):1935–45.
Cheng VK, Au PC, Tan KC, Cheung CL. MicroRNA and human bone health. JBMR Plus. 2019;3(1):2–13.
Phetfong J, Sanvoranart T, Nartprayut K, Nimsanor N, Seenprachawong K, Prachayasittikul V, et al. Osteoporosis: the current status of mesenchymal stem cell-based therapy. Cell Mol Biol Lett. 2016;21:12.
Li J, Lu L, Liu Y, Yu X. Bone marrow adiposity during pathologic bone loss: molecular mechanisms underlying the cellular events. J Mol Med (Berl). 2022;100(2):167–83.
Song H, Li X, Zhao Z, Qian J, Wang Y, Cui J, et al. Reversal of osteoporotic activity by endothelial cell-secreted bone targeting and biocompatible exosomes. Nano Lett. 2019;19(5):3040–8.
Kusumbe AP, Ramasamy SK, Adams RH. Coupling of angiogenesis and osteogenesis by a specific vessel subtype in bone. Nature. 2014;507(7492):323–8.
Xu R, Yallowitz A, Qin A, Wu Z, Shin DY, Kim JM, et al. Targeting skeletal endothelium to ameliorate bone loss. Nat Med. 2018;24(6):823–33.
Chen H, Hu B, Lv X, Zhu S, Zhen G, Wan M, et al. Prostaglandin E2 mediates sensory nerve regulation of bone homeostasis. Nat Commun. 2019;10(1):181.
Seely KD, Kotelko CA, Douglas H, Bealer B, Brooks AE. The human gut microbiota: a key mediator of osteoporosis and osteogenesis. Int J Mol Sci. 2021;22(17):9452.
De Martinis M, Ginaldi L, Allegra A, Sirufo MM, Pioggia G, Tonacci A, et al. The osteoporosis/microbiota linkage: the role of miRNA. Int J Mol Sci. 2020;21(23):8887.
Liu JH, Chen CY, Liu ZZ, Luo ZW, Rao SS, Jin L, et al. Extracellular vesicles from child gut microbiota enter into bone to preserve bone mass and strength. Adv Sci (Weinh). 2021;8(9):2004831.
Xie H, Cui Z, Wang L, Xia Z, Hu Y, Xian L, et al. PDGF-BB secreted by preosteoclasts induces angiogenesis during coupling with osteogenesis. Nat Med. 2014;20(11):1270–8.
Weske S, Vaidya M, Reese A, von Wnuck LK, Keul P, Bayer JK, et al. Targeting sphingosine-1-phosphate lyase as an anabolic therapy for bone loss. Nat Med. 2018;24(5):667–78.
Qiu Z, Li L, Huang Y, Shi K, Zhang L, Huang C, et al. Puerarin specifically disrupts osteoclast activation via blocking integrin-beta3 Pyk2/Src/Cbl signaling pathway. J Orthop Translat. 2022;33:55–69.
Zhu M, Yin P, Hu F, Jiang J, Yin L, Li Y, et al. Integrating genome-wide association and transcriptome prediction model identifies novel target genes for osteoporosis. Osteoporos Int. 2021;32(12):2493–503.
Mo XB, Zhang YH, Lei SF. Genome-wide identification of m(6)A-associated SNPs as potential functional variants for bone mineral density. Osteoporos Int. 2018;29(9):2029–39.
Komori T. Animal models for osteoporosis. Eur J Pharmacol. 2015;759:287–94.
Cui J, Shibata Y, Zhu T, Zhou J, Zhang J. Osteocytes in bone aging: advances, challenges, and future perspectives. Ageing Res Rev. 2022;77: 101608.
Song S, Guo Y, Yang Y, Fu D. Advances in pathogenesis and therapeutic strategies for osteoporosis. Pharmacol Ther. 2022;237: 108168.
Khosla S, Farr JN, Monroe DG. Cellular senescence and the skeleton: pathophysiology and therapeutic implications. J Clin Invest. 2022;132(3).
Mazziotti G, Lania AG, Canalis E. Skeletal disorders associated with the growth hormone–insulin-like growth factor 1 axis. Nat Rev Endocrinol. 2022;18(6):353–65.
Soós B, Szentpétery Á, Raterman HG, Lems WF, Bhattoa HP, Szekanecz Z. Effects of targeted therapies on bone in rheumatic and musculoskeletal diseases. Nat Rev Rheumatol. 2022;18(5):249–57.
Zhu K, Prince RL. Calcium and bone. Clin Biochem. 2012;45(12):936–42.
Pludowski P, Holick MF, Grant WB, Konstantynowicz J, Mascarenhas MR, Haq A, et al. Vitamin D supplementation guidelines. J Steroid Biochem Mol Biol. 2018;175:125–35.
Yao P, Bennett D, Mafham M, Lin X, Chen Z, Armitage J, et al. Vitamin D and calcium for the prevention of fracture: a systematic review and meta-analysis. JAMA Netw Open. 2019;2(12): e1917789.
Hou YC, Wu CC, Liao MT, Shyu JF, Hung CF, Yen TH, et al. Role of nutritional vitamin D in osteoporosis treatment. Clin Chim Acta. 2018;484:179–91.
Alshahrani F, Aljohani N. Vitamin D: deficiency, sufficiency and toxicity. Nutrients. 2013;5(9):3605–16.
Chiodini I, Bolland MJ. Calcium supplementation in osteoporosis: useful or harmful? Eur J Endocrinol. 2018;178(4):D13-d25.
Center JR, Lyles KW, Bliuc D. Bisphosphonates and lifespan. Bone. 2020;141: 115566.
Hermann AP, Abrahamsen B. The bisphosphonates: risks and benefits of long term use. Curr Opin Pharmacol. 2013;13(3):435–9.
Ilyas Z, Camacho PM. Rare adverse effects of bisphosphonate therapy. Curr Opin Endocrinol Diabetes Obes. 2019;26(6):335–8.
Bluming AZ, Tavris C. Hormone replacement therapy: real concerns and false alarms. Cancer J. 2009;15(2):93–104.
Lobo RA. Hormone-replacement therapy: current thinking. Nat Rev Endocrinol. 2017;13(4):220–31.
Liu JH. Selective estrogen receptor modulators (SERMS): keys to understanding their function. Menopause. 2020;27(10):1171–6.
Palacios S, Brincat M, Erel CT, Gambacciani M, Lambrinoudaki I, Moen MH, et al. EMAS clinical guide: selective estrogen receptor modulators for postmenopausal osteoporosis. Maturitas. 2012;71(2):194–8.
Pinkerton JV, Conner EA. Beyond estrogen: advances in tissue selective estrogen complexes and selective estrogen receptor modulators. Climacteric. 2019;22(2):140–7.
Brommage R. New targets and emergent therapies for osteoporosis. Handb Exp Pharmacol. 2020;262:451–73.
Tanaka S, Yoshida A, Kono S, Ito M. Effectiveness of monotherapy and combined therapy with calcitonin and minodronic acid hydrate, a bisphosphonate, for early treatment in patients with new vertebral fractures: an open-label, randomized, parallel-group study. J Orthop Sci. 2017;22(3):536–41.
Yazdani J, Khiavi RK, Ghavimi MA, Mortazavi A, Hagh EJ, Ahmadpour F. Calcitonin as an analgesic agent: review of mechanisms of action and clinical applications. Braz J Anesthesiol. 2019;69(6):594–604.
Dai R, Wu Z, Chu HY, Lu J, Lyu A, Liu J, et al. Cathepsin K: the action in and beyond bone. Front Cell Dev Biol. 2020;8:433.
Drake MT, Clarke BL, Oursler MJ, Khosla S. Cathepsin K inhibitors for osteoporosis: biology, potential clinical utility, and lessons learned. Endocr Rev. 2017;38(4):325–50.
Stone JA, McCrea JB, Witter R, Zajic S, Stoch SA. Clinical and translational pharmacology of the cathepsin K inhibitor odanacatib studied for osteoporosis. Br J Clin Pharmacol. 2019;85(6):1072–83.
McClung MR, O’Donoghue ML, Papapoulos SE, Bone H, Langdahl B, Saag KG, et al. Odanacatib for the treatment of postmenopausal osteoporosis: results of the LOFT multicentre, randomised, double-blind, placebo-controlled trial and LOFT Extension study. Lancet Diabetes Endocrinol. 2019;7(12):899–911.
Leder BZ. Optimizing sequential and combined anabolic and antiresorptive osteoporosis therapy. JBMR Plus. 2018;2(2):62–8.
Lamy O, Stoll D, Aubry-Rozier B, Rodriguez EG. Stopping denosumab. Curr Osteoporos Rep. 2019;17(1):8–15.
Augustine M, Horwitz MJ. Parathyroid hormone and parathyroid hormone-related protein analogs as therapies for osteoporosis. Curr Osteoporos Rep. 2013;11(4):400–6.
Fan Y, Hanai JI, Le PT, Bi R, Maridas D, DeMambro V, et al. Parathyroid hormone directs bone marrow mesenchymal cell fate. Cell Metab. 2017;25(3):661–72.
Osagie-Clouard L, Sanghani A, Coathup M, Briggs T, Bostrom M, Blunn G. Parathyroid hormone 1–34 and skeletal anabolic action. Bone Joint Res. 2017;6(1):14–21.
Anastasilakis AD, Polyzos SA, Yavropoulou MP, Makras P. Combination and sequential treatment in women with postmenopausal osteoporosis. Expert Opin Pharmacother. 2020;21(4):477–90.
Delgado-Calle J, Sato AY, Bellido T. Role and mechanism of action of sclerostin in bone. Bone. 2017;96:29–37.
Fixen C, Tunoa J. Romosozumab: a review of efficacy, safety, and cardiovascular risk. Curr Osteoporos Rep. 2021;19(1):15–22.
Saag KG, Petersen J, Brandi ML, Karaplis AC, Lorentzon M, Thomas T, et al. Romosozumab or alendronate for fracture prevention in women with osteoporosis. N Engl J Med. 2017;377(15):1417–27.
Kołodziejska B, Stępień N, Kolmas J. The influence of strontium on bone tissue metabolism and its application in osteoporosis treatment. Int J Mol Sci. 2021;22(12):6564.
Saidak Z, Marie PJ. Strontium signaling: molecular mechanisms and therapeutic implications in osteoporosis. Pharmacol Ther. 2012;136(2):216–26.
Pilmane M, Salma-Ancane K, Loca D, Locs J, Berzina-Cimdina L. Strontium and strontium ranelate: historical review of some of their functions. Mater Sci Eng C Mater Biol Appl. 2017;78:1222–30.
Abrahamsen B, Grove EL, Vestergaard P. Nationwide registry-based analysis of cardiovascular risk factors and adverse outcomes in patients treated with strontium ranelate. Osteoporos Int. 2014;25(2):757–62.
Reginster JY, Brandi ML, Cannata-Andía J, Cooper C, Cortet B, Feron JM, et al. The position of strontium ranelate in today’s management of osteoporosis. Osteoporos Int. 2015;26(6):1667–71.
Arjmand B, Sarvari M, Alavi-Moghadam S, Payab M, Goodarzi P, Gilany K, et al. Prospect of stem cell therapy and regenerative medicine in osteoporosis. Front Endocrinol (Lausanne). 2020;11:430.
Volarevic V, Markovic BS, Gazdic M, Volarevic A, Jovicic N, Arsenijevic N, et al. Ethical and safety issues of stem cell-based therapy. Int J Med Sci. 2018;15(1):36–45.
Anita Sanghani-Kerai DM, Lancashire H, Osagie L, Coathup M, Blunn G. Stem cell interventions for bone healing fractures and osteoporosis. Curr Stem Cell Res Therapy. 2018;13(5):369–77.
Chen HT, Lee MJ, Chen CH, Chuang SC, Chang LF, Ho ML, et al. Proliferation and differentiation potential of human adipose-derived mesenchymal stem cells isolated from elderly patients with osteoporotic fractures. J Cell Mol Med. 2012;16(3):582–93.
Liu HY, Chiou JF, Wu AT, Tsai CY, Leu JD, Ting LL, et al. The effect of diminished osteogenic signals on reduced osteoporosis recovery in aged mice and the potential therapeutic use of adipose-derived stem cells. Biomaterials. 2012;33(26):6105–12.
Hsiao FS, Cheng CC, Peng SY, Huang HY, Lian WS, Jan ML, et al. Isolation of therapeutically functional mouse bone marrow mesenchymal stem cells within 3 h by an effective single-step plastic-adherent method. Cell Prolif. 2010;43(3):235–48.
Ye X, Zhang P, Xue S, Xu Y, Tan J, Liu G. Adipose-derived stem cells alleviate osteoporosis by enhancing osteogenesis and inhibiting adipogenesis in a rabbit model. Cytotherapy. 2014;16(12):1643–55.
Cho SW, Sun HJ, Yang JY, Jung JY, An JH, Cho HY, et al. Transplantation of mesenchymal stem cells overexpressing RANK-Fc or CXCR4 prevents bone loss in ovariectomized mice. Mol Ther. 2009;17(11):1979–87.
Götherström C, Westgren M, Shaw SW, Aström E, Biswas A, Byers PH, et al. Pre- and postnatal transplantation of fetal mesenchymal stem cells in osteogenesis imperfecta: a two-center experience. Stem Cells Transl Med. 2014;3(2):255–64.
Taiani JT, Buie HR, Campbell GM, Manske SL, Krawetz RJ, Rancourt DE, et al. Embryonic stem cell therapy improves bone quality in a model of impaired fracture healing in the mouse; tracked temporally using in vivo micro-CT. Bone. 2014;64:263–72.
Yu Z, Zhu T, Li C, Shi X, Liu X, Yang X, et al. Improvement of intertrochanteric bone quality in osteoporotic female rats after injection of polylactic acid-polyglycolic acid copolymer/collagen type I microspheres combined with bone mesenchymal stem cells. Int Orthop. 2012;36(10):2163–71.
Havens AM, Shiozawa Y, Jung Y, Sun H, Wang J, McGee S, et al. Human very small embryonic-like cells generate skeletal structures, in vivo. Stem Cells Dev. 2013;22(4):622–30.
Aggarwal R, Lu J, Kanji S, Joseph M, Das M, Noble GJ, et al. Human umbilical cord blood-derived CD34 + cells reverse osteoporosis in NOD/SCID mice by altering osteoblastic and osteoclastic activities. PLoS ONE. 2012;7(6): e39365.
You L, Pan L, Chen L, Chen JY, Zhang X, Lv Z, et al. Suppression of zinc finger protein 467 alleviates osteoporosis through promoting differentiation of adipose derived stem cells to osteoblasts. J Transl Med. 2012;10:11.
Zhang C, Hu K, Liu X, Reynolds MA, Bao C, Wang P, et al. Novel hiPSC-based tri-culture for pre-vascularization of calcium phosphate scaffold to enhance bone and vessel formation. Mater Sci Eng C Mater Biol Appl. 2017;79:296–304.
Hadjiargyrou M, Komatsu DE. The therapeutic potential of microRNAs as orthobiologics for skeletal fractures. J Bone Miner Res. 2019;34(5):797–809.
Inoue K, Nakano S, Zhao B. Osteoclastic microRNAs and their translational potential in skeletal diseases. Semin Immunopathol. 2019;41(5):573–82.
Dou C, Ding N, Luo F, Hou T, Cao Z, Bai Y, et al. Graphene-based microRNA transfection blocks preosteoclast fusion to increase bone formation and vascularization. Adv Sci (Weinh). 2021;8(15): e2102286.
Lima JF, Cerqueira L, Figueiredo C, Oliveira C, Azevedo NF. Anti-miRNA oligonucleotides: a comprehensive guide for design. RNA Biol. 2018;15(3):338–52.
Krützfeldt J, Rajewsky N, Braich R, Rajeev KG, Tuschl T, Manoharan M, et al. Silencing of microRNAs in vivo with ‘antagomirs.’ Nature. 2005;438(7068):685–9.
Ebert MS, Neilson JR, Sharp PA. MicroRNA sponges: competitive inhibitors of small RNAs in mammalian cells. Nat Methods. 2007;4(9):721–6.
Liu HP, Hao DJ, Wang XD, Hu HM, Li YB, Dong XH. miR-30a-3p promotes ovariectomy-induced osteoporosis in rats via targeting SFRP1. Eur Rev Med Pharmacol Sci. 2019;23(22):9754–60.
Shi C, Wu T, He Y, Zhang Y, Fu D. Recent advances in bone-targeted therapy. Pharmacol Ther. 2020;207: 107473.
Zheng L, Zhuang Z, Li Y, Shi T, Fu K, Yan W, et al. Bone targeting antioxidative nano-iron oxide for treating postmenopausal osteoporosis. Bioact Mater. 2022;14:250–61.
Cui Y, Guo Y, Kong L, Shi J, Liu P, Li R, et al. A bone-targeted engineered exosome platform delivering siRNA to treat osteoporosis. Bioact Mater. 2022;10:207–21.
Download references
Acknowledgements
We thank Shuting Lin and Wenjin Guo for their help in revising the paper.
This work was supported by the Science and Technology Bureau of Quanzhou (Grant#2020CT003) to SL, Nursery fund of the Second Affiliated Hospital of Fujian Medical University (2020BSH04) to BL, Science and Technology Plan Project of Quanzhou (Grant#2021N033S) to BL, and the National Health & Medical Research Council, Australia (NH&MRC, #1162276) to Y-CS.
Author information
Shu Lin and Yan-Chuan Shi are joint senior authors.
Authors and Affiliations
Department of Endocrinology and Metabolism, The Second Affiliated Hospital of Fujian Medical University, Quanzhou, China
Neuroendocrinology Group, Garvan Institute of Medical Research, 384 Victoria Street, Darlinghurst, Sydney, NSW, 2010, Australia
George Burley, Shu Lin & Yan-Chuan Shi
Centre of Neurological and Metabolic Research, The Second Affiliated Hospital of Fujian Medical University, No.34 North Zhongshan Road, Quanzhou, 362000, Fujian Province, China
Shu Lin & Yan-Chuan Shi
St Vincent’s Clinical School, Faculty of Medicine, UNSW Sydney, Sydney, Australia
Yan-Chuan Shi
You can also search for this author in PubMed Google Scholar
Contributions
B.L. conducted literature review and drafted the original manuscript. S.L., Y.-C.S., and G.B. revised and reviewed the manuscript. All authors read and approved the final manuscript.
Corresponding authors
Correspondence to Shu Lin or Yan-Chuan Shi .
Ethics declarations
Ethics approval and consent to participate, consent for publication.
All authors agree to publish.
Competing interests
The authors declare that they have no conflicts of interest.
Additional information
Publisher’s note.
Springer Nature remains neutral with regard to jurisdictional claims in published maps and institutional affiliations.
Rights and permissions
Open Access This article is licensed under a Creative Commons Attribution 4.0 International License, which permits use, sharing, adaptation, distribution and reproduction in any medium or format, as long as you give appropriate credit to the original author(s) and the source, provide a link to the Creative Commons licence, and indicate if changes were made. The images or other third party material in this article are included in the article's Creative Commons licence, unless indicated otherwise in a credit line to the material. If material is not included in the article's Creative Commons licence and your intended use is not permitted by statutory regulation or exceeds the permitted use, you will need to obtain permission directly from the copyright holder. To view a copy of this licence, visit http://creativecommons.org/licenses/by/4.0/ .
Reprints and permissions
About this article
Cite this article.
Liang, B., Burley, G., Lin, S. et al. Osteoporosis pathogenesis and treatment: existing and emerging avenues. Cell Mol Biol Lett 27 , 72 (2022). https://doi.org/10.1186/s11658-022-00371-3
Download citation
Received : 02 April 2022
Accepted : 09 August 2022
Published : 04 September 2022
DOI : https://doi.org/10.1186/s11658-022-00371-3
Share this article
Anyone you share the following link with will be able to read this content:
Sorry, a shareable link is not currently available for this article.
Provided by the Springer Nature SharedIt content-sharing initiative
- Osteoporosis
- Pathogenesis
- Bone remodeling
- Bone formation
- Bone resorption
- MicroRNA-based therapy
Cellular & Molecular Biology Letters
ISSN: 1689-1392
- Submission enquiries: Access here and click Contact Us
- General enquiries: [email protected]
Osteoporosis
The information on this page has been compiled from the research presented in the videos listed. Sources for each video can be found by going to the video’s page and clicking on the Sources Cited tab.
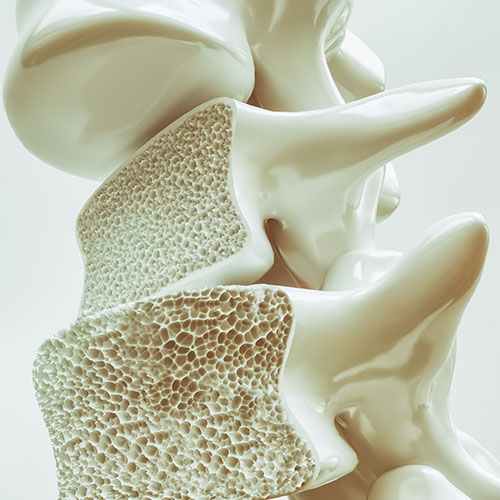
Subscribe to our free newsletter and receive the preface of Dr. Greger’s upcoming book How Not to Age .
Popular Videos for Osteoporosis
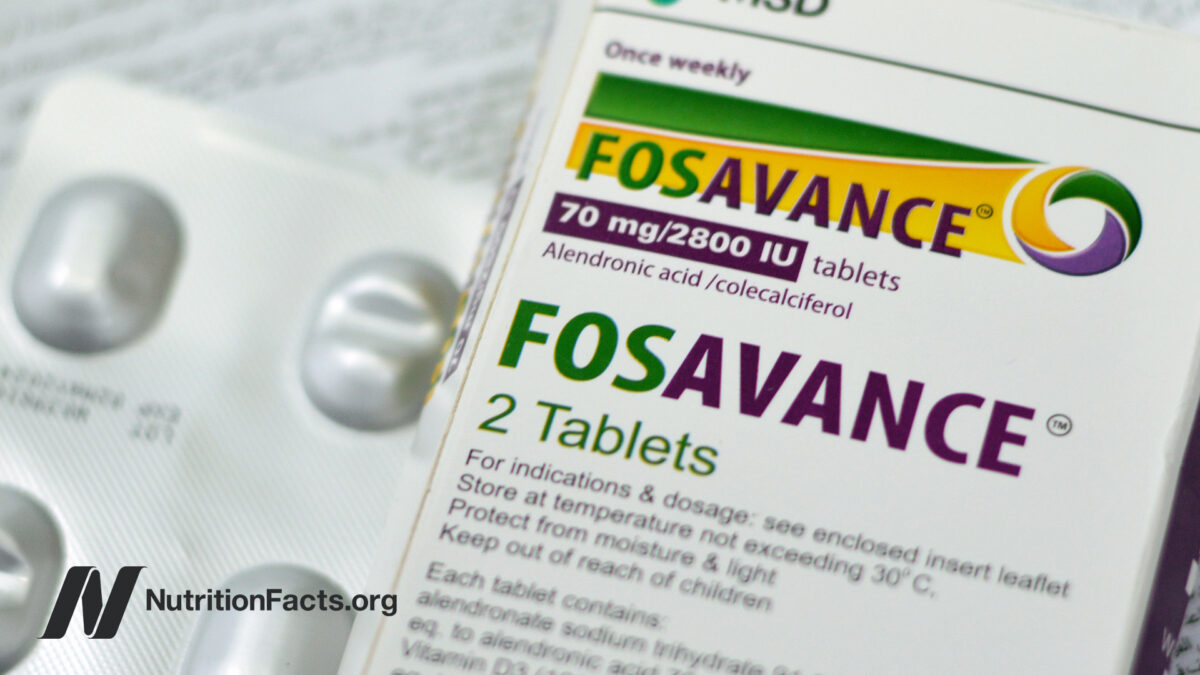
Side Effects of Osteoporosis Medications Like Fosamax, Boniva, and Reclast
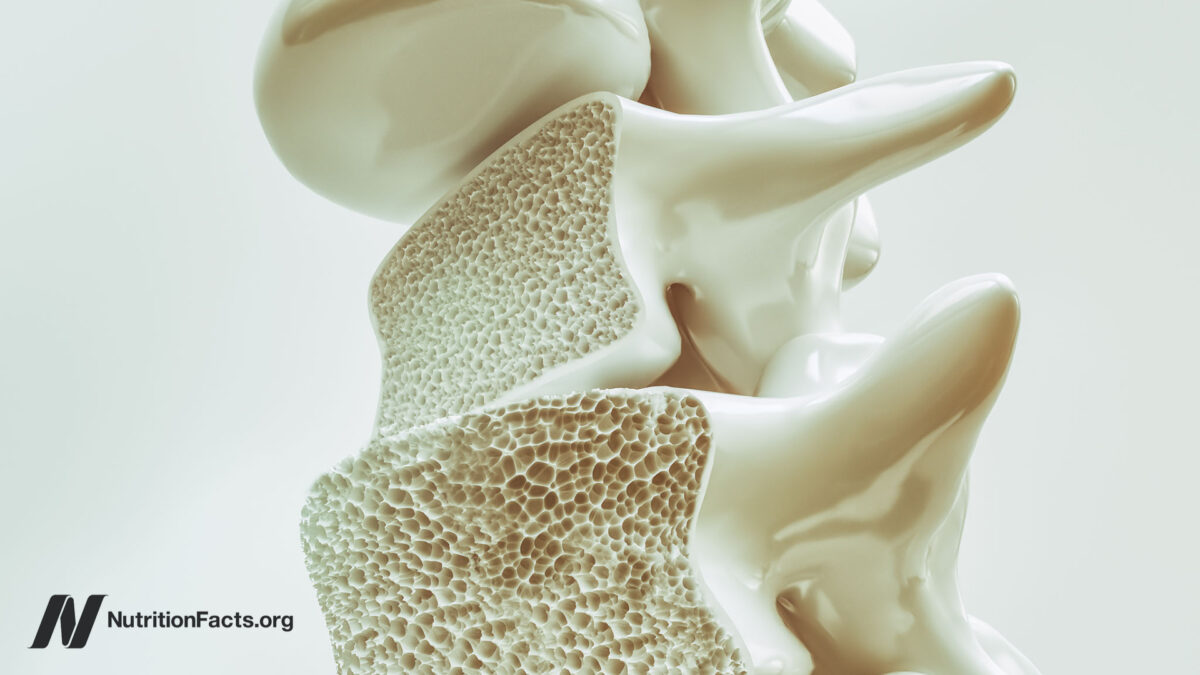
How Well Do Medicines Like Fosamax Work to Treat Osteoporosis?
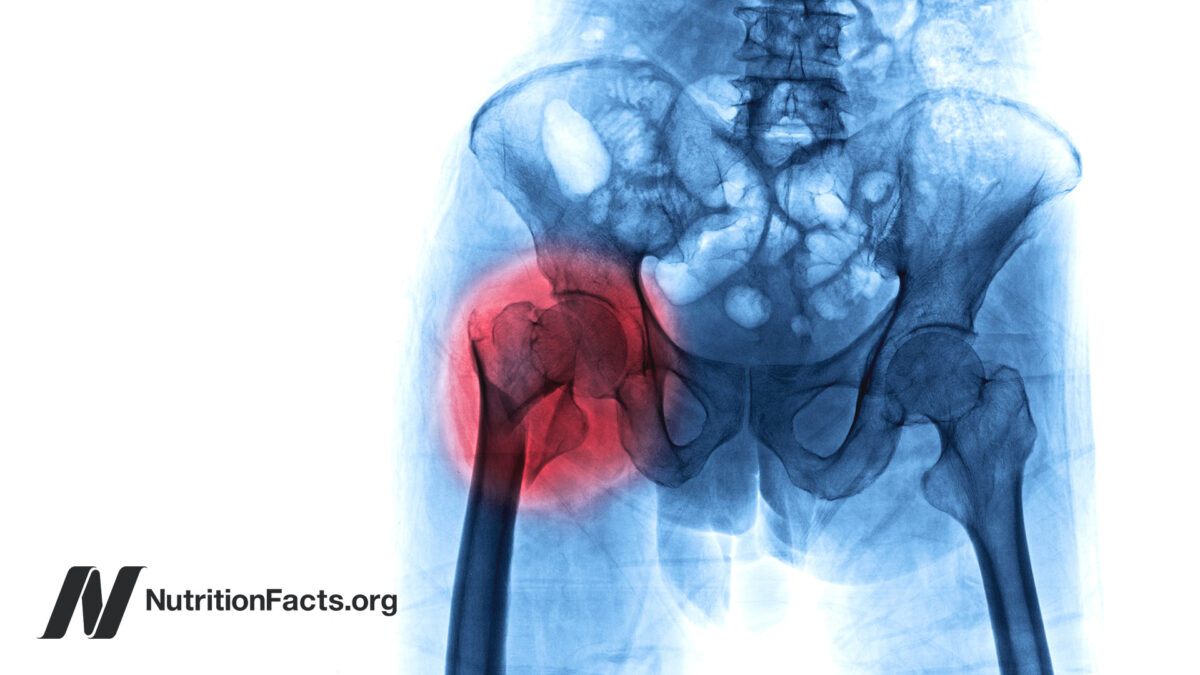
Acid Reflux Medicine May Cause Osteoporosis
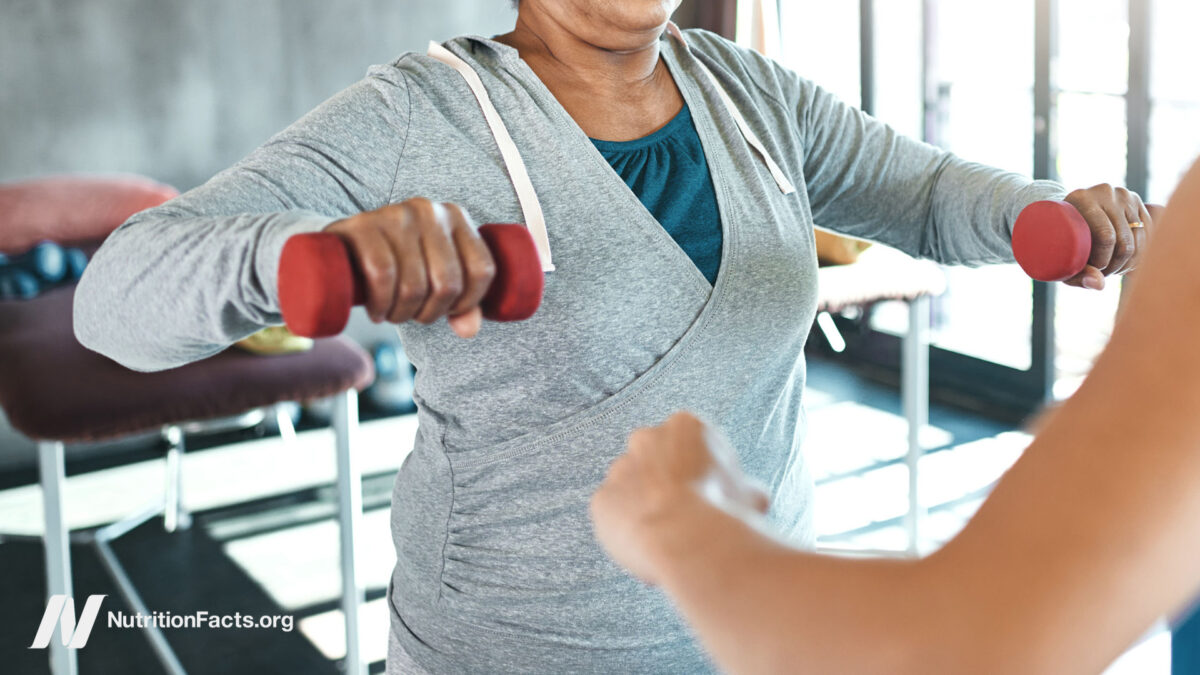
Fall Prevention Is the Most Important Thing for Preventing Osteoporosis Bone Fractures
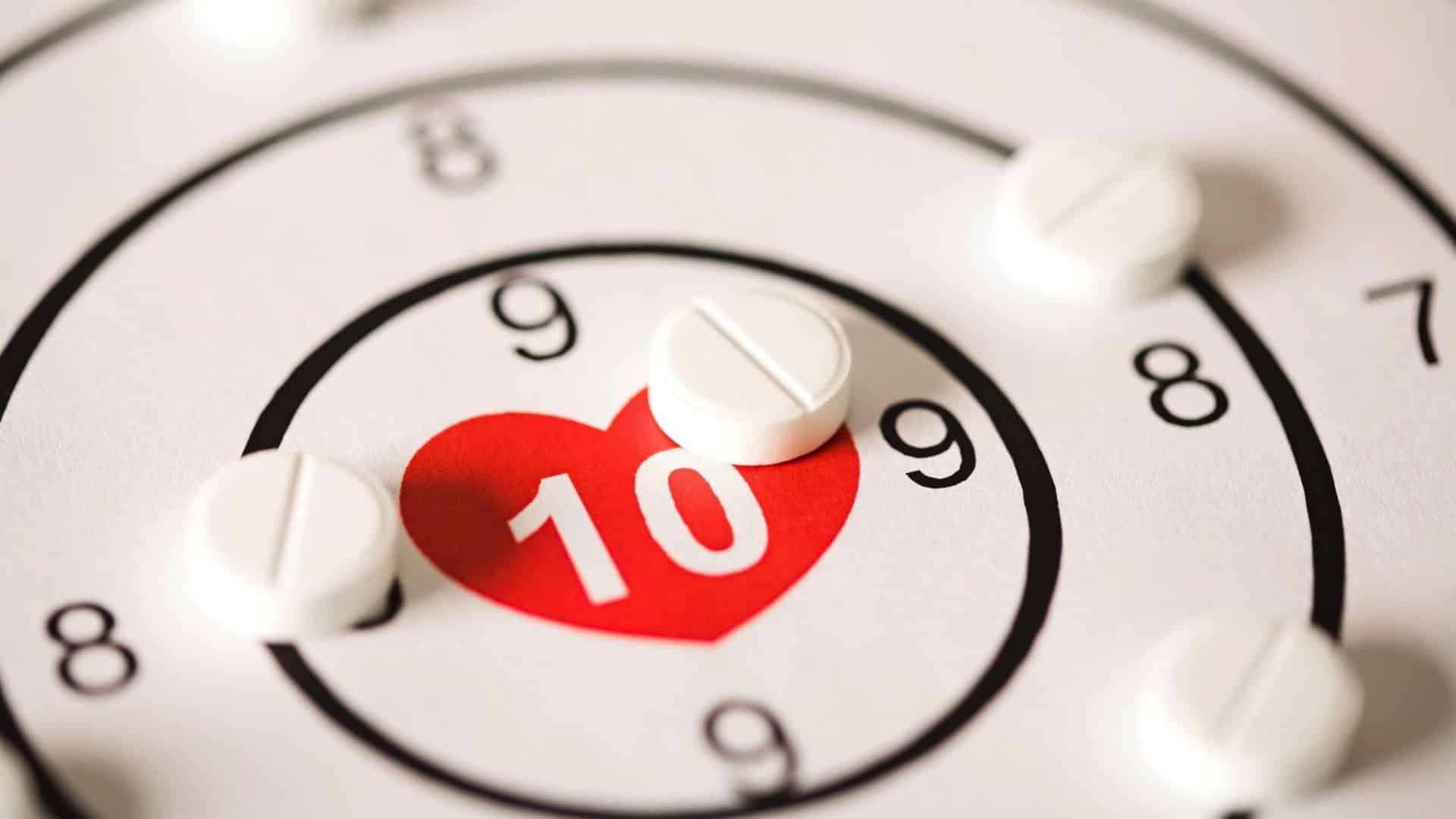
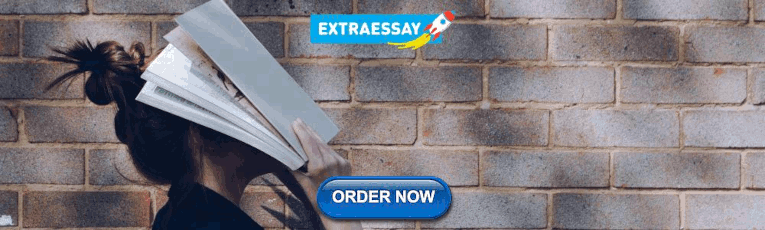
Are Calcium Supplements Safe?
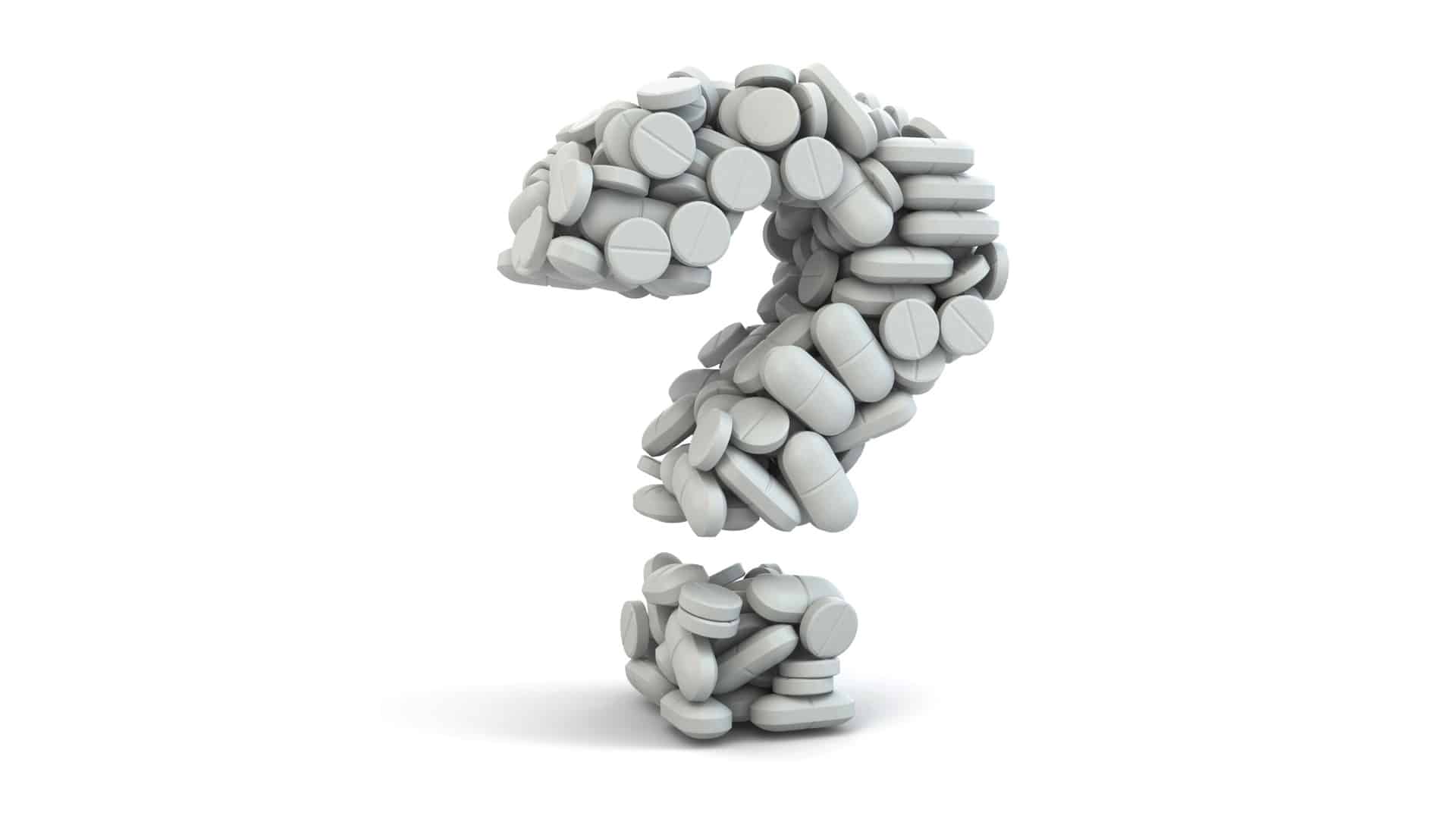
Are Calcium Supplements Effective?
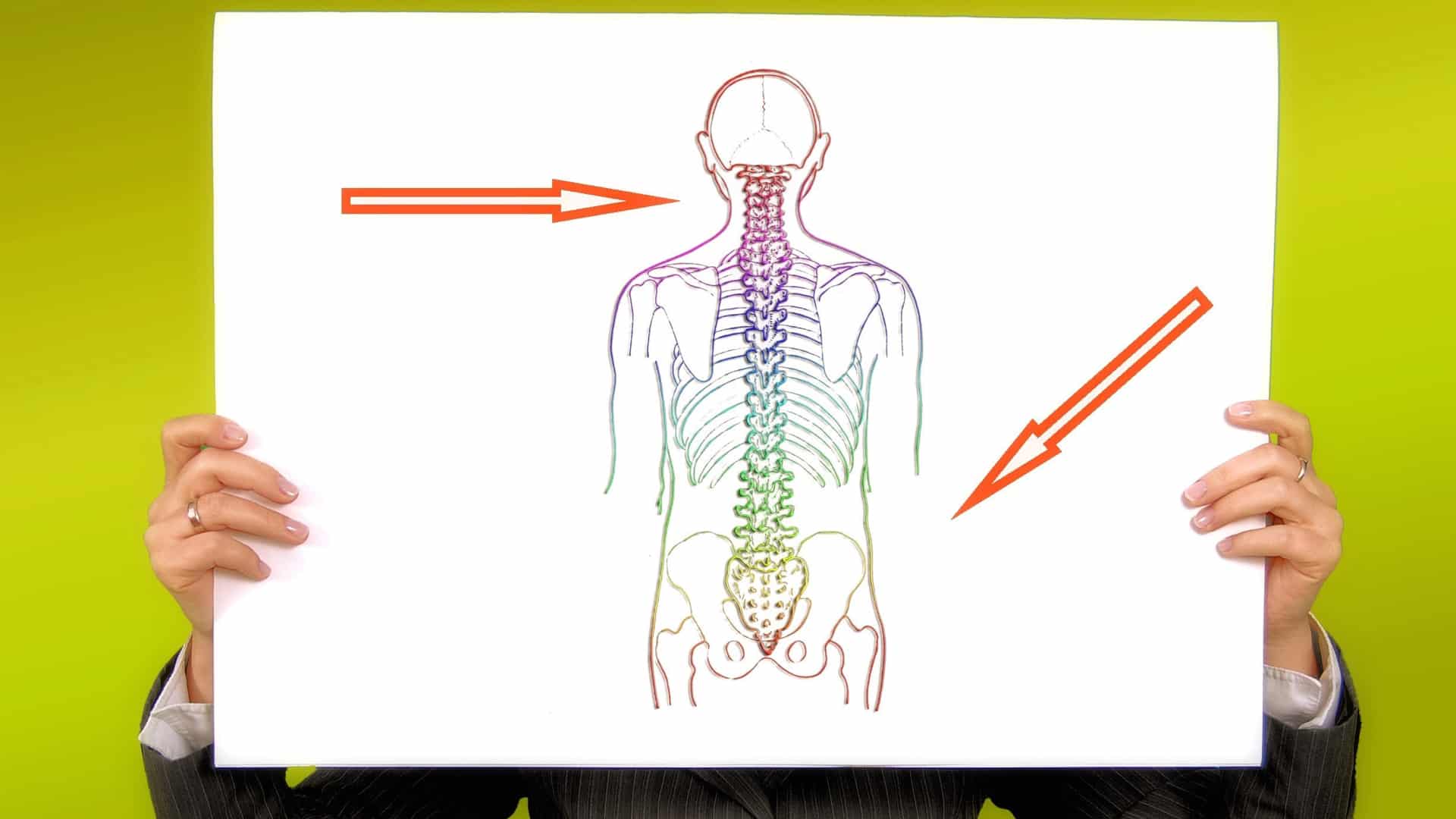
Prunes for Osteoporosis
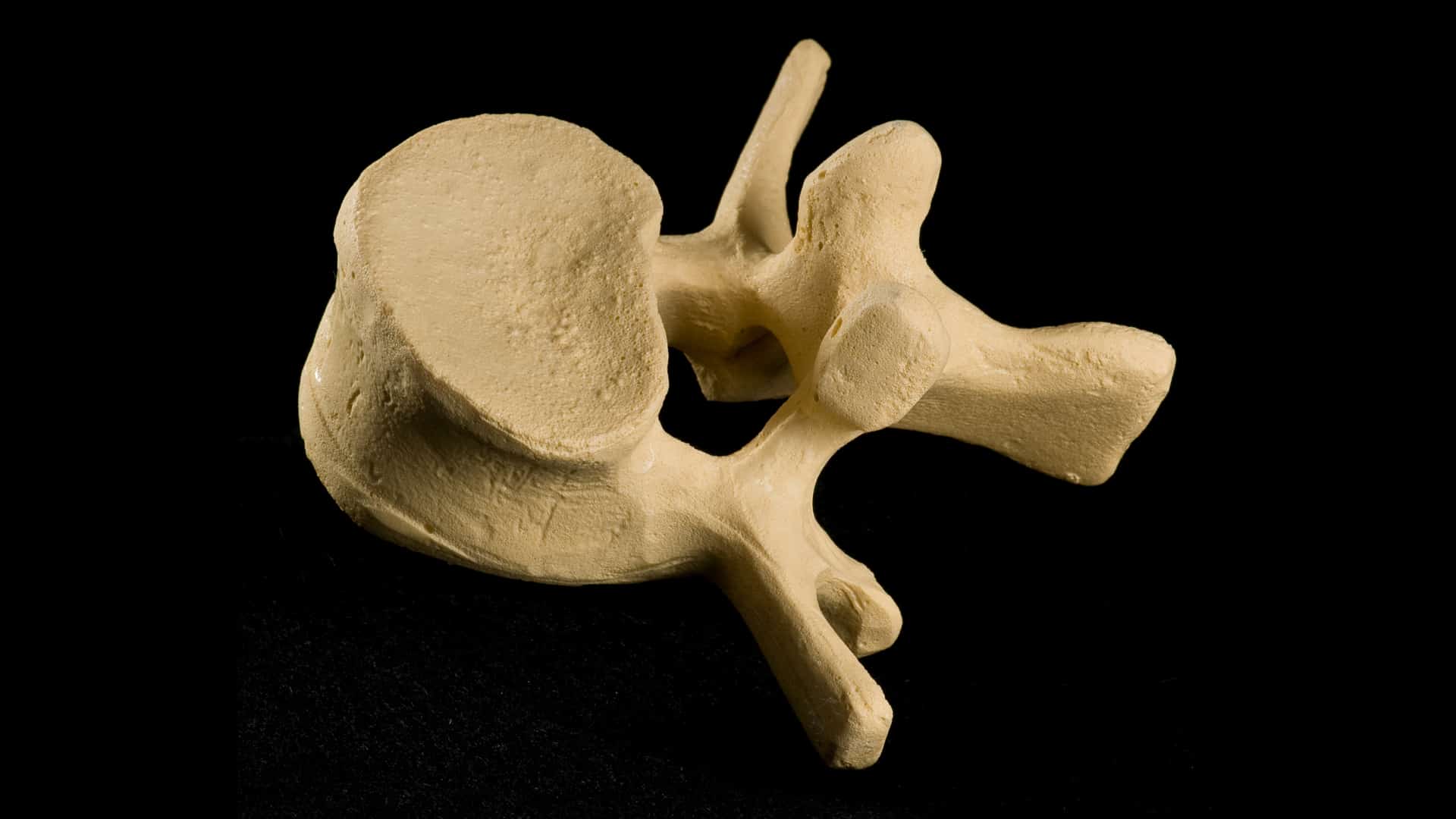
Almonds for Osteoporosis
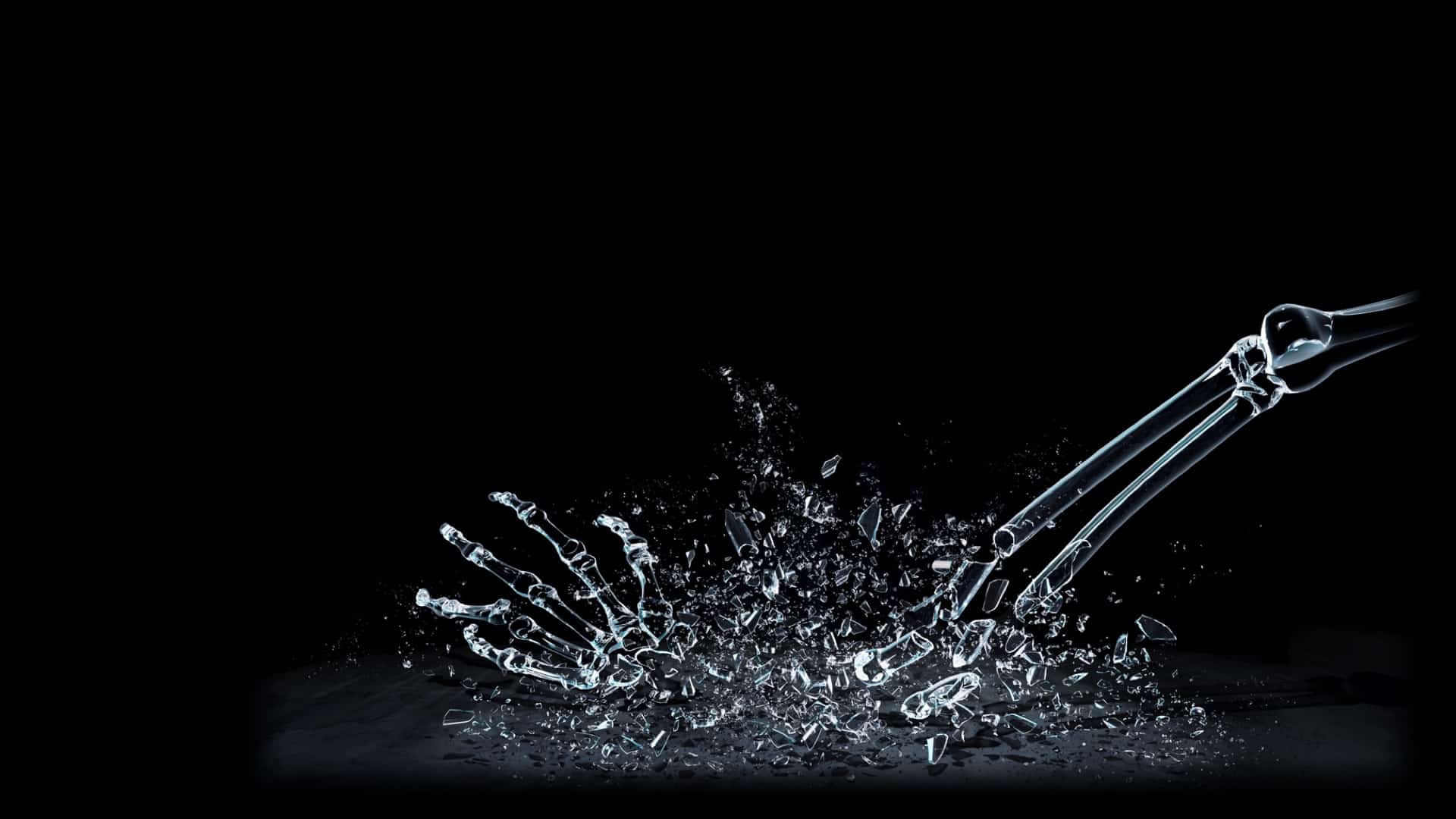
Is Milk Good for Our Bones?
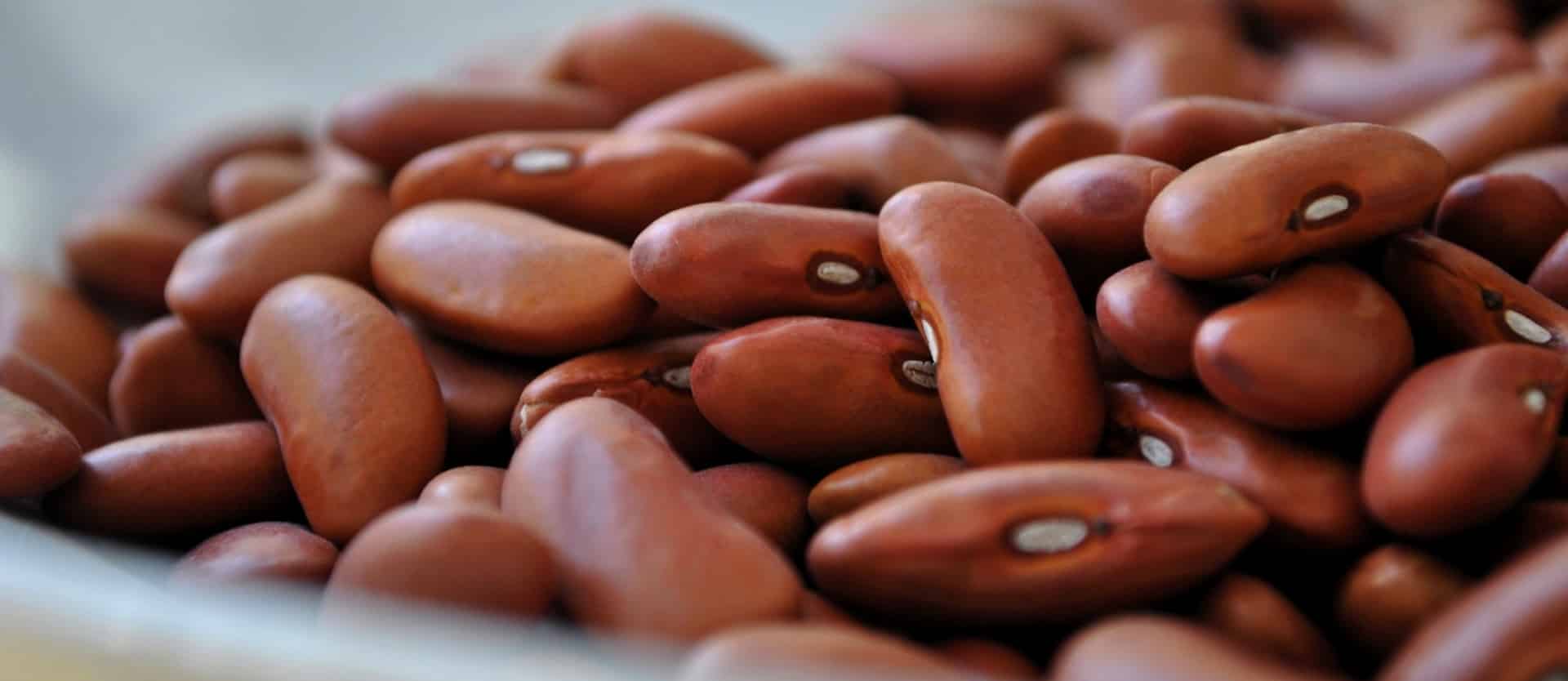
Phytates for the Prevention of Osteoporosis
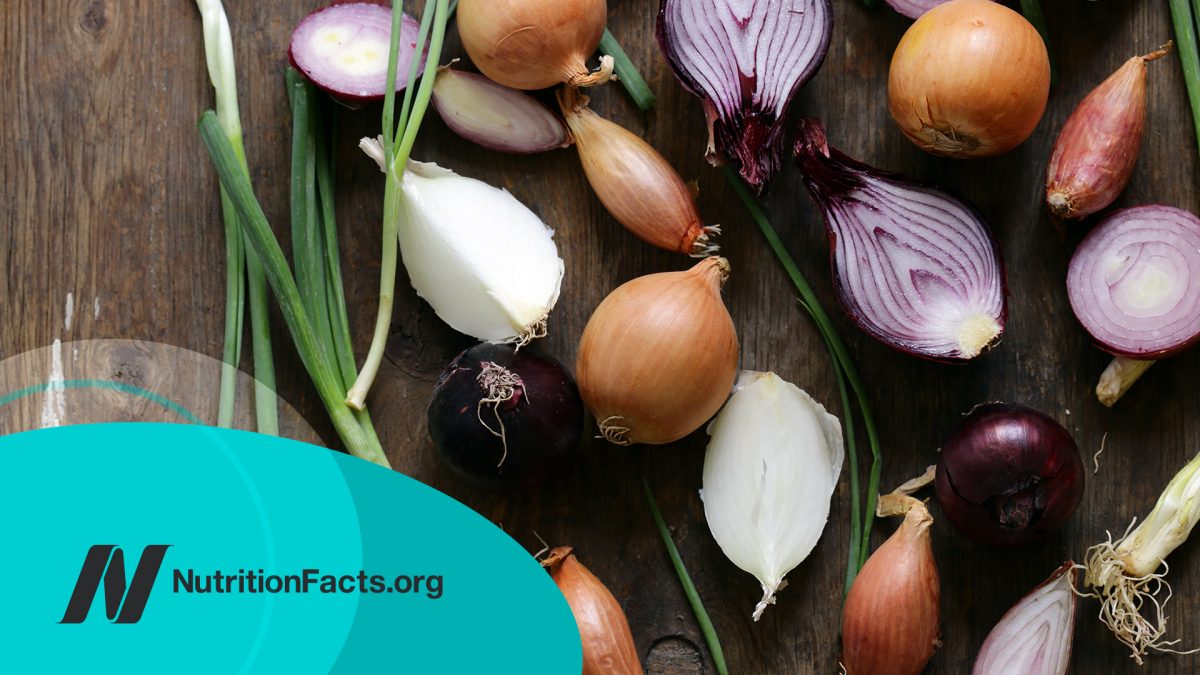
Are Onions Beneficial for Testosterone, Osteoporosis, Allergies, and Cancer?
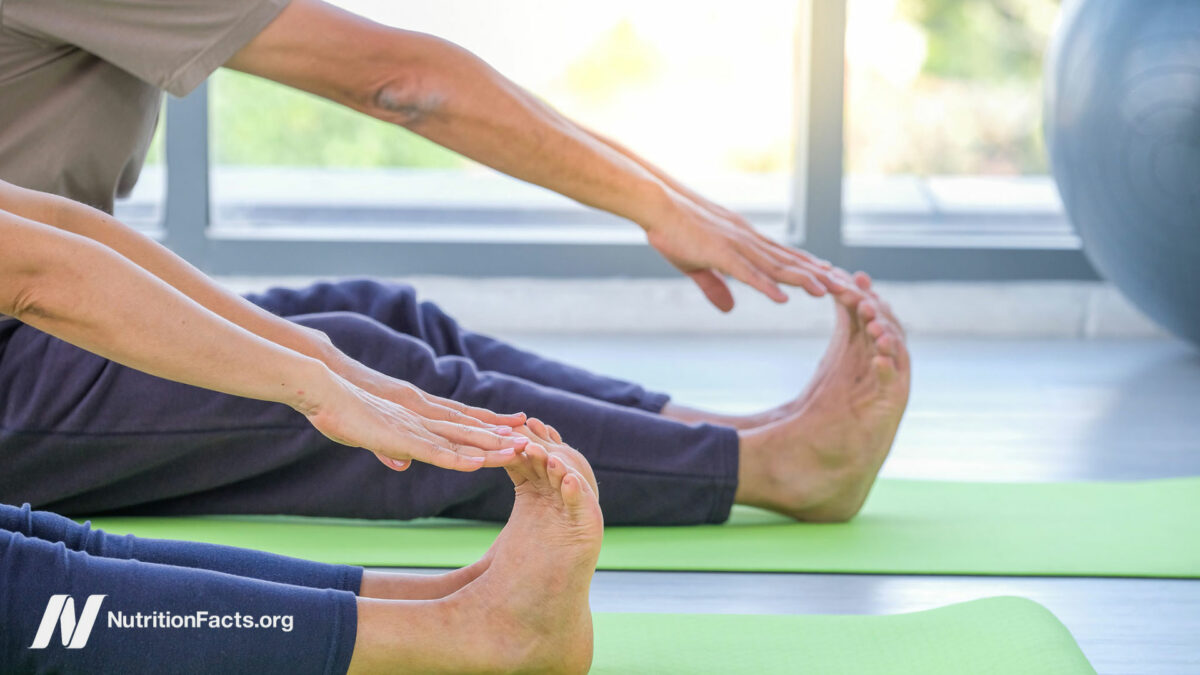
Yoga Put to the Test for IBS, Inflammatory Bowel, Menopause, and Osteoporosis
All videos for osteoporosis.
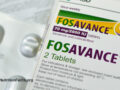
How rare are the bisphosphonate class of osteoporosis drugs’ devastating side effects, which ironically include bone fractures?
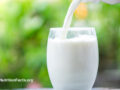
Why Do Milk Drinkers Live Shorter Lives on Average?
How might we reduce the risk of premature death from dairy consumption?
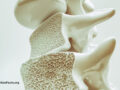
Doctors and patients alike vastly overestimate the power of bisphosphonate drugs to prevent fractures.
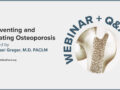
Preventing and Treating Osteoporosis (Webinar Recording)
I’m happy to share the recording of my webinar on osteoporosis, which includes 11 videos and a Q&A.
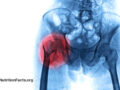
Stomach acid–blocking proton pump inhibitor drugs—PPIs with brand names like Prilosec, Prevacid, Nexium, Protonix, and AcipHex—appear to significantly increase the risk of bone fractures.
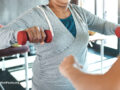
A combination of resistance exercise to improve lower limb muscle strength and balance training can beat out drugs for preventing osteoporotic bone fractures.
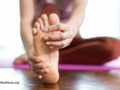
The Side Effects of Yoga
What is the rate of yoga injuries compared to other activities?
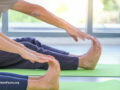
A study using sham acupuncture underscores the necessity of controlling for expectancy effects.
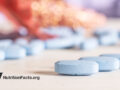
Vitamin D May Explain Higher Bone Fracture Risk in Vegans
A combination of low calcium intake and low vitamin D exposure may explain higher bone fracture rates in British vegans.
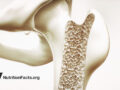
Do Vegans Have Lower Bone Density and More Fractures?
What are the bone fracture rates of omnivores vs. vegetarians vs. vegans?
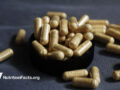
The Purported Benefits of Vitamin K2: Should You Take Supplements?
Our body can make vitamin K2 from the K1 in green leafy vegetables.
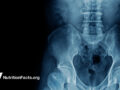
Do Vegans Have Lower Bone Mineral Density and Higher Risk of Osteoporosis?
Those eating plant-based tend to be so much slimmer that their bone mass may suffer.
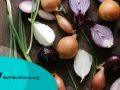
What did randomized controlled human trials find about the ways we may—or may not—benefit from eating onions?
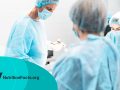
The Complications of Bariatric Weight-Loss Surgery
The extent of risk from bariatric weight-loss surgery may depend on the skill of the surgeon.
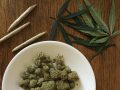
Effects of Marijuana on Weight Gain and Bone Density
Are the apparent adverse effects of heavy cannabis use on bone just due to users being skinnier?
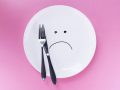
Potential Pitfalls of Calorie Restriction
How to preserve bone and mass on a low calorie diet.
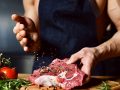
Keto Diets: Muscle Growth and Bone Density
Ketogenic diets found to undermine exercise efforts and lead to muscle shrinkage and bone loss.
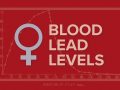
The Rise in Blood Lead Levels at Pregnancy and Menopause
The lead trapped in our skeleton can leach back into our bloodstream when we temporarily or permanently lose bone due to pregnancy, weight loss, menopause, or osteoporosis.
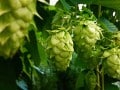
What Are the Effects of the Hops Phytoestrogen in Beer?
When it comes to breast cancer risk, does the phytoestrogen in beer act more like the animal estrogens in Premarin or the protective phytoestrogens in soy?
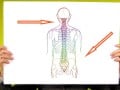
Vegetables and fruit, such as dried plums, may help build stronger bones.
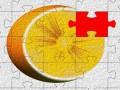
Reductionism and the Deficiency Mentality
How the food, drug, and supplement industries have taken advantage of the field of nutrition’s reductionist mindset
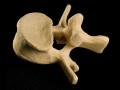
What happens when you take blood from people before and then again four hours after almond consumption, and drip that blood on bone cells?
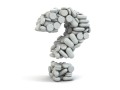
What is the optimal daily dietary calcium intake and might benefits for your bones outweigh the risks to your heart from taking calcium supplements?
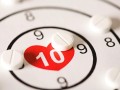
The unnaturally large, rapid, and sustained calcium levels in the blood caused by calcium supplements may explain why calcium from supplements, but not from food, appears to increase the risk of heart attacks.
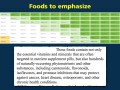
What Are the Healthiest Foods?
Based on the Dietary Guidelines for Americans, which foods best supply shortfall nutrients while avoiding disease-promoting components?
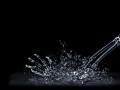
The galactose in milk may explain why milk consumption is associated with significantly higher risk of hip fractures, cancer, and premature death.
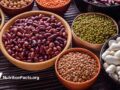
Beans, Beans, They’re Good for Your Heart
Legumes such as lentils, chickpeas, beans and split peas may reduce cholesterol so much that consumers may be able to get off their cholesterol-lowering statin drugs, but to profoundly alter heart disease risk we may have to more profoundly alter our diet.
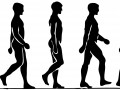
Longer Life Within Walking Distance
Researchers find exercise often works just as well as drugs for the treatment of heart disease and stroke, and the prevention of diabetes. Exercise is medicine.
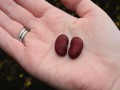
Phytates for the Treatment of Cancer
Do the anticancer effects of phytates in a petri dish translate out into clinical studies on cancer prevention and treatment?
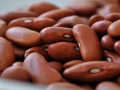
Women who consume the most high-phytate foods (whole grains, beans, and nuts) appear to have better bone density.
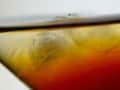
Phosphate Additives in Meat Purge and Cola
The consumption of phosphorus preservatives in junk food, and injected into meat, may damage blood vessels, accelerate the aging process, and contribute to osteoporosis.
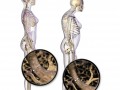
Alkaline Diets, Animal Protein, and Calcium Loss
The decades-old dogma that the acid-forming quality of animal protein leads to bone loss has been called into question.
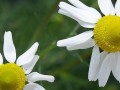
Chamomile Tea May Not Be Safe During Pregnancy
For the same reason aspirin should be avoided in pregnancy, chamomile has such powerful anti-inflammatory properties that regular consumption may result in a serious fetal heart problem—premature constriction of the fetal ductus arteriosus, which allows the fetus to “breathe” in the womb.
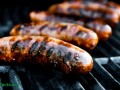
Glycotoxins
Advanced glycation end products (AGEs) in our diet are thought to accelerate the aging process.
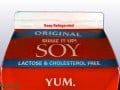
Calcium Absorption: Soy Milk Versus Cow Milk
Soy milk should be shaken before pouring to get at the calcium that settles to the bottom.
Pin It on Pinterest
Eating More Eggs Might Help Protect Against Osteoporosis, Research Shows
Fact checked by Nick Blackmer
- New research has found a link between eating eggs and improved bone density, which indicates a lower risk of osteoporosis.
- Egg consumption could improve the body’s production of a specific enzyme that leads to stronger bones, experts said.
- This and other research suggests that eating one to two eggs daily could help people boost their bone and heart health.
Your morning egg scramble could be doing more than simply fueling you until lunch—it might also be strengthening your skeleton.
A new study found whole egg consumption is related to greater bone mineral density in the U.S. population. The research was published in January in Food and Function.
Calcium-rich foods such as leafy greens and dairy products have long held top billing as the healthiest choices for bones, but they’re far from the only foods that support a solid skeleton. This new research could cement eggs as yet another dietary option to reduce the risk of osteoporosis (weak bones).
“This is not the first study that linked egg consumption with bone health,” study author Weihong Chen, MD , chief of the Department of Occupational and Environmental Health at Huazhong University of Science and Technology, told Health .
A preprint scoping review published in October called for more evidence on the subject but said eggs may be a way to boost bone density and lower fracture risk among older people. Additional research, such as a 2021 study in the Journal of Midlife Health , has also identified a relationship between eating whole eggs and having sturdier bones, Chen pointed out.
However, Chen and her colleagues’ study may have more impact than previous ones since, to her knowledge, it involved more participants.
Here’s what experts had to say about the new study, as well as how eggs may be able to help you protect against osteoporosis.
Investigating the Connection Between Eggs and Bone Density
To learn more about how a diet rich in eggs might affect people's bone health, Chen and her colleagues designed a study including over 19,000 people who had participated in the National Health and Nutrition Examination Survey. This longitudinal study has been in existence since the early 1960s, but this study used data collected from 2005–2010, 2013–2014, and 2017–2018.
Researchers had access to these participants’ bone mineral density (BMD), as well as their survey results regarding egg consumption. The team’s analysis revealed participants who consumed at least 3.53 ounces of whole eggs daily—about two large eggs—had significantly elevated BMD levels in their femurs and spines.
Bone mineral density measures calcium and other minerals in the bones. Low BMD is a sign of osteoporosis—when bones are less dense, they’re more likely to break.
Older people are at a higher risk of developing osteoporosis since, as we age, we lose more bone than we build. In particular, older women may be more likely to get the condition because estrogen levels (which help build and maintain healthy bones) drop after menopause .
But age and gender aren’t the only factors playing a role in a person’s osteoporosis risk. Insufficient nutrition, low levels of physical activity, smoking, drinking excessive amounts of alcohol, and long-term use of certain drugs such as corticosteroids can all put a person at risk of weak, brittle bones.
Why Eggs Might Be Able to Keep Bones Healthy
Eggs are well-known for being a low-calorie breakfast option, as well as for containing a moderate amount of protein (about 6 grams per large chicken egg). However, people might not turn to eggs as a way to protect their bone health—after all, eggs are not rich in calcium, containing just 24 milligrams, or about 2% of adults’ recommended daily intake.
But according to the new Food and Function study, eggs appear to activate a group of bodily enzymes called alkaline phosphatase, which can strengthen bones.
“Alkaline phosphatase (ALP) is a group of enzymes mainly existing in the liver, bones, kidneys, etc., which is a biomarker of bone metabolism and is not a part of the egg,” Chen explained. “The consumption of whole eggs could affect the production of ALP, which significantly affects the bone mineral density of both femur and lumbar spine.”
The researchers found that ALP’s role accounted for about 72% of eggs’ effect on bone density in the femur and 83% in the lumbar spine.
“[This suggests] that a large part of the benefits of egg consumption on these bone areas is because of how they impact ALP levels,” said Chen.
Besides this enzymatic effect, eggs are also rich in several nutrients that support healthy bones.
“Eggs contain vitamin D , which helps your body absorb calcium, a mineral that is essential for strong bones,” Kathryn Piper, RDN , registered dietician nutritionist and founder of The Age-Defying Dietitian, told Health . “Additionally, eggs are packed with protein, zinc, and other minerals that contribute to overall bone health.”
In fact, the protein in eggs is another possible reason for their bone-building abilities.
“Eggs are an excellent source of protein, and previous studies have indicated the indispensable role of protein in the calcium and phosphorus metabolism, vitamin transport, and bone remodeling balance,” said Chen.
Egg protein even contains amino acid sequences called bioactive peptides that may have extra benefits for bones, she noted.
Related: 11 Foods for Strengthening Bones
Incorporating Eggs Into Your Diet
For years, discussion around the healthfulness of eggs has been fraught with concerns about their leading to high cholesterol or cardiovascular disease. However, research suggests eggs can be part of a heart-healthy diet, and the American Heart Association even encourages Americans to eat eggs daily as a source of high-quality protein.
“Studies suggest that moderate egg consumption (around one to two whole eggs daily) doesn’t significantly impact cholesterol levels in healthy individuals,” Piper added.
People with pre-existing high cholesterol should discuss their egg intake with their doctor, said Piper, but in general, eating about two eggs daily seems to boost both heart and bone health.
When incorporating eggs into a healthy diet, just remember that the way you cook them matters.
“Boil, poach, or lightly scramble eggs with minimal oil,” Piper suggested. “These cooking methods maximize the nutritional benefits of eggs without adding unhealthy fats.”
Additionally, what you put with eggs can add to or detract from the healthfulness of a meal.
“For a more balanced approach, combine eggs with nutrient-rich foods like vegetables, whole grains, and fruits,” she added. “Think veggie-filled omelets, avocado toast, salads, or whole-wheat sandwiches featuring eggs.”
Related: The Healthiest Way to Prepare an Egg
For more Health.com news, make sure to sign up for our newsletter!
Read the original article on Health.com .
- Inside Griffith
- Find an expert
- Media contacts
- Student blog
- Architecture, construction and planning
- Business and government
- Criminology and law
- Engineering, IT and aviation
- Humanities and languages
- International
- Music and performing arts
- Science and environment
- Sustainability
- Visual and creative arts
- Staff Portal
- Griffith News
- Sustainable Development
- 3 Good Health and Well Being
Referral pathway study for osteoporosis patients
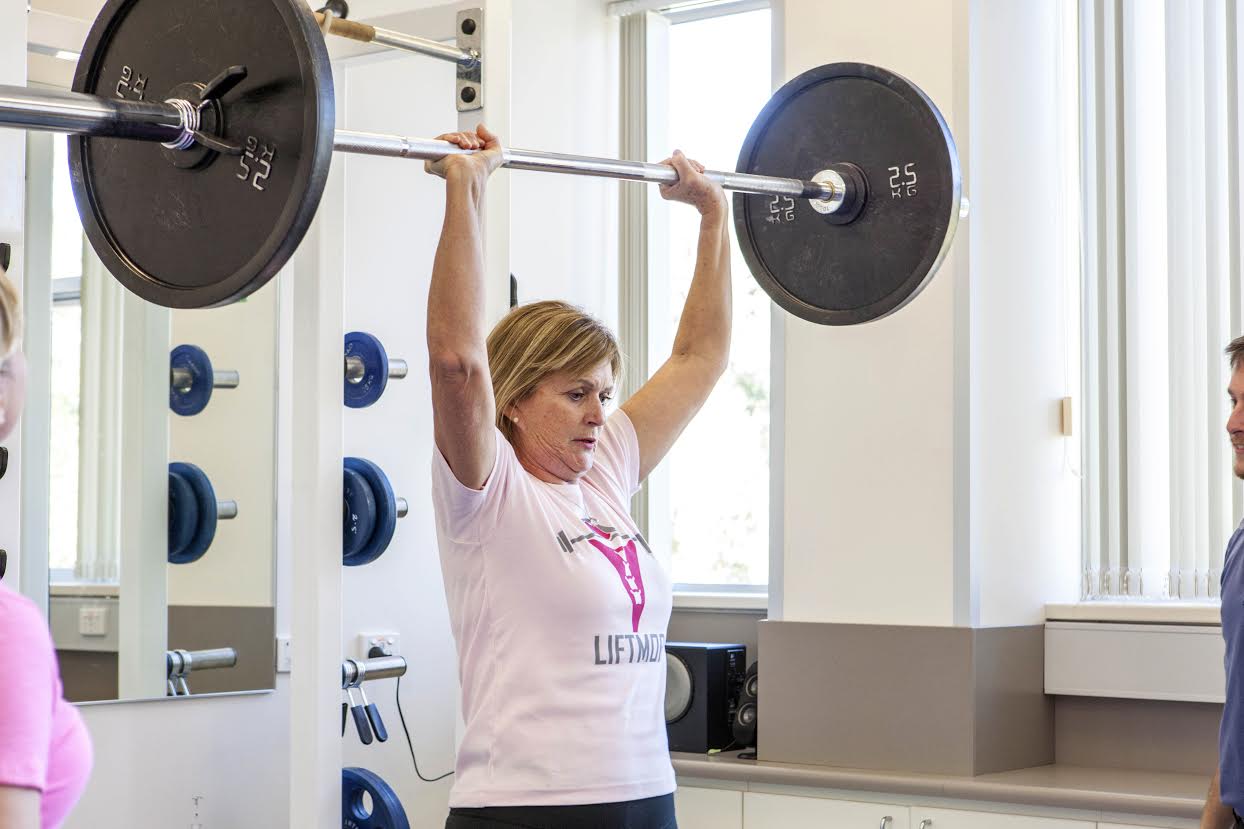
Clinicians are being encouraged to refer patients with osteoporosis to a heavy lifting exercise program to improve bone health and reduce the risk of fragility fractures which can occur from minimal trauma such as a fall.
Those fractures can dramatically reduce quality of life, lead to a loss of independence, and increase risk of an earlier death.
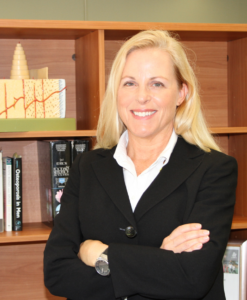
Professor Belinda Beck and her research team of Dr Amy Harding and Julian Currie in Griffith University’s School of Health Sciences and Social Work are conducting the STOP FRACTURE! study, aiming to reduce the number of fragility fractures in Australians over the age 45.
“Osteoporosis is a silent epidemic and unfortunately most people don’t even know they have low bone mass until they fracture doing something innocuous,” Professor Beck said.
“Our study is looking at upgrading the osteoporosis model of care to include referral to an exercise program that we have shown, in clinical trials here at Griffith, builds bone and improves muscle strength and other risk factors for fracture.
“The exercise involves lifting weights, which doctors tend to avoid because people with osteoporosis can be quite frail, but we have found it is safe and effective if supervised closely.”
The goal of the STOP FRACTURE! study is to increase doctor awareness of this effective exercise program, called Onero, for osteoporosis and to determine whether this greater awareness increases referrals and prevents fragility fractures.
The Bone Clinic is a translational research clinic established to implement Onero into clinical practice with ongoing patient monitoring to determine if it is safe and effective in the real world.
Nearly nine years on, the results speak for themselves.
“We have patients in their 20s to those in their 90s lifting weights and the effect is always incredible,” Professor Beck said.
“This program has a strong emphasis on prevention because we know once you’ve had your first fracture, you’re four times more likely to have another.
“But we have lots of people lifting weights who have already had multiple fractures, and they are going great guns too.
“In the STOP FRACTURE! study, we’re working closely with doctors in hospitals and medical practices to establish a sustainable referral pathway so we can continue to help people to prevent fractures into the future.”
Professor Beck received a $1.4 million MRFF grant for her STOP FRACTURE! Project, which stands for Strength Training for Optimum Prevention of Fracture: Refocussing a Clinical Paradigm that Underutilises Recognised Effective Therapy.
More information on the program can be found here .
Plan encourages habitat protection to prevent pandemics
Qld disaster research alliance appoints alliance manager, sports engineering student proves a key player in paralympic innovation, persian plateau unveiled as crucial hub for early human migration out of africa.
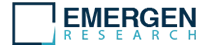
Top 10 Companies in Osteoporosis Drugs Market in 2024
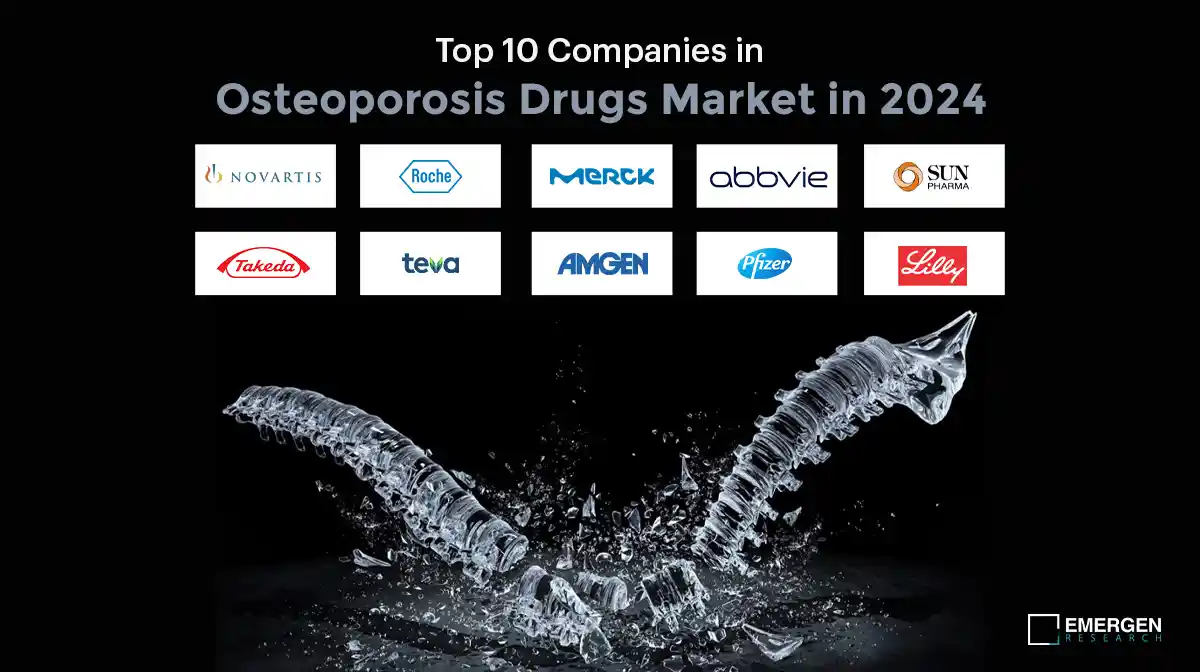
Introduction
In the realm of osteoporosis treatment, certain pharmaceutical juggernauts have carved their names into the annals of healthcare history. These companies, driven by innovation, research prowess, and a commitment to patient well-being, stand at the forefront of battling this pervasive bone disease. Join us as we unveil the top 10 osteoporosis drugs companies in the world, delving into their remarkable contributions, groundbreaking achievements, and the impact they've had on reshaping the landscape of osteoporosis treatment.
Osteoporosis drugs are medications used to treat osteoporosis, a condition characterized by weakened bones prone to fractures. These drugs work by slowing bone loss or promoting bone formation. They include bisphosphonates, hormone replacement therapy, selective estrogen receptor modulators, calcitonin, RANK ligand inhibitors, parathyroid hormone analogs, and monoclonal antibodies . Treatment is tailored based on factors like age, gender, and medical history, often combined with lifestyle changes like exercise and calcium supplementation to strengthen bones and reduce fracture risk.
The global osteoporosis drugs market is anticipated to witness significant revenue growth driven by the continuous launch of more effective medications and increasing investment in research and development by the pharmaceutical industry. However, market expansion may be restrained by factors such as drug side effects, patent expirations, regulatory hurdles, and lengthy FDA approval processes. Despite the growing prevalence of osteoporosis and efforts to introduce new therapeutic alternatives, challenges such as adverse reactions and stringent regulations pose obstacles to sustained market growth.
The global osteoporosis medications market size was worth USD 13.06 billion in 2022, and it is predicted to grow at a 4.7% CAGR over the forecast period. The rising frequency of osteoporosis diseases, as well as an increase in senior populations, are two main reasons driving revenue growth in the osteoporosis medications market.
World’s Prominent Companies Offering Osteoporosis Drugs; Top 10 by Revenue
- Novartis AG
- Hoffmann-La Roche Ltd
- Sun Pharmaceutical Industries Ltd
- Takeda Pharmaceutical Company Limited
- Teva Pharmaceutical Industries Ltd.
- Pfizer Inc.
Top 10 Globally Leading Companies in The Osteoporosis Drugs Market
Pfizer inc. [annual revenue: usd 61 billion].
Pfizer Inc. , a renowned pharmaceutical giant founded in 1849, boasts a diverse portfolio of medications across various therapeutic areas. In the realm of osteoporosis, Pfizer offers key drugs like Forteo, Xeljanz, and Duavee, catering to the treatment and prevention of bone loss. Notable for breakthroughs like Viagra and Prevnar 13, Pfizer continues its pioneering research, collaborating and conducting clinical trials to address unmet medical needs in osteoporosis management. With a commitment to innovation and global reach, Pfizer remains a leader in providing effective solutions for patients worldwide.
Hoffmann-La Roche Ltd [Annual Revenue: USD 58.7 Billion]
Hoffmann-La Roche Ltd is a leading pharmaceutical company with a rich history dating back to 1896. Renowned for its innovation in healthcare, Roche specializes in developing and manufacturing a wide range of pharmaceuticals, including breakthrough treatments for osteoporosis such as Boniva (ibandronate) and Evista (raloxifene). Notable achievements include pioneering advancements in biotechnology and personalized medicine. Recent developments involve ongoing research and development efforts to enhance osteoporosis treatment efficacy and patient outcomes, aligning with Roche's commitment to addressing unmet medical needs and improving global health.
AbbVie [Annual Revenue: USD 54.32 Billion]
AbbVie , a leading pharmaceutical company established in 2013, is dedicated to discovering, developing, and delivering innovative therapies across various therapeutic areas, including osteoporosis. While its primary focus spans immunology, oncology, neuroscience, virology, and women's health, AbbVie's commitment to research and development extends to osteoporosis treatments, with potential key products like Durolane, Orilissa, and Parathyroid Hormone (PTH) Analogs. Known for its innovation, patient-centric approach, global reach, and collaborative partnerships, AbbVie aims to address the unmet needs of patients with osteoporosis, contributing to improved bone health and overall well-being.
Novartis AG [Annual Revenue: USD 46.66 Billion]
Novartis AG , a Swiss multinational pharmaceutical company founded in 1996, boasts a rich history of innovation and excellence in the pharmaceutical industry. In the osteoporosis drugs market, Novartis is notable for its development of Denosumab, marketed as Prolia®, offering effective treatment for postmenopausal women and men at high risk of fractures. Continuously investing in research and development, Novartis maintains a strong commitment to addressing unmet medical needs and improving patient outcomes, positioning itself as a key player in the global healthcare landscape.
Lilly [Annual Revenue: USD 41.6 Billion]
Lilly, or Eli Lilly and Company , is a leading pharmaceutical firm with a history dating back to 1876, renowned for its focus on osteoporosis treatment. Key products like Forteo and Evista offer innovative solutions, increasing bone density and reducing fracture risk. Recent developments likely involve advancements in drug delivery and formulation. With a reputation for quality and a global reach, Lilly remains a pivotal player in the osteoporosis drugs market, committed to enhancing patient outcomes through ongoing research and innovation.
Takeda Pharmaceutical Company Limited [Annual Revenue: USD 29.795 Billion]
Takeda Pharmaceutical Company Limited , founded in 1781 and headquartered in Tokyo, Japan, is a global leader in the pharmaceutical industry. With a comprehensive portfolio spanning various therapeutic areas, including osteoporosis treatment, Takeda offers key products like Actonel and Miacalcin. Notable for its commitment to innovation, Takeda conducts clinical trials and forges partnerships to advance osteoporosis care. With a presence in over 80 countries, Takeda ensures global access to quality treatments, solidifying its position as a leading player in the osteoporosis drugs market.
Amgen Inc. [Annual Revenue: USD 28.19 Billion]
Amgen Inc. is a leading biotechnology company renowned for its innovative therapies. Established in 1980, Amgen has made significant contributions to healthcare, offering a diverse portfolio of products targeting various medical conditions. In the osteoporosis drugs market, notable offerings include Prolia and Evenity, which have revolutionized treatment options for postmenopausal women at high risk of fractures. With a commitment to research and development, Amgen continues to advance biotechnology, delivering impactful solutions to improve patients' lives worldwide.
Merck KGaA [Annual Revenue: USD 23.38 Billion]
Merck KGaA , a multinational pharmaceutical and life sciences company, is renowned in the osteoporosis drugs market for Binosto® (alendronic acid), an effervescent tablet formulation of alendronate, aiding in bone density enhancement and fracture prevention in osteoporosis patients. With a rich history dating back to 1668, Merck KGaA's commitment to innovation and patient care shines through its convenient drug delivery systems and ongoing research efforts, ensuring it remains a key player in providing effective treatments for osteoporosis worldwide.
Teva Pharmaceutical Industries Ltd. [Annual Revenue: USD 16.3 Billion]
Teva Pharmaceutical Industries Ltd . is a global leader in generic and specialty pharmaceuticals, offering a diverse portfolio of affordable medications across various therapeutic areas, including osteoporosis treatment. With a history dating back to 1901, Teva has expanded its focus to include innovative drug development and biopharmaceuticals. Notable achievements include market leadership, a broad distribution network, and strategic partnerships. Recent developments highlight Teva's commitment to sustainability and its efforts to address unmet medical needs. In the osteoporosis drugs market, Teva's extensive portfolio of generic medications provides cost-effective options, contributing to improved access to treatment worldwide.
Sun Pharmaceutical Industries Ltd [Annual Revenue: USD 5.6 Billion]
Sun Pharmaceutical Industries Ltd. is a multinational pharmaceutical company founded in 1983, headquartered in Mumbai, India. It specializes in generic and specialty pharmaceuticals, active pharmaceutical ingredients (APIs), and research and development. With a presence in over 100 countries, Sun Pharma is a global leader known for its diverse portfolio, regulatory approvals, successful acquisitions, and focus on research and innovation. In the osteoporosis drugs market, Sun Pharma develops and markets generic and specialty formulations, contributing to its position as a prominent player in the global pharmaceutical industry.
Please support us by following us on LinkedIn - https://www.linkedin.com/company/emergen-research
Read Comprehensive Report
Latest blogs.
Looking for Customization?
Have a Question?
Any Confusion?
Have a glance of the Report

How can we help you?
Please contact for a detailed synopsis of the report
Research Report
- Chemicals and Advanced Materials 511
- Healthcare and Pharmaceuticals 929
- Power and Energy 57
- Foods & Beverage 89
- Automotive and Transportation 134
- Information Technology 544
- Manufacturing and Construction 57
- Semiconductors & Electronics 334
- Consumer Goods 50
- Aerospace and Defense 134
Service Guarantee

Order And Delivery
This report has a service guarantee. We stand by our report qualityDelivery within 48 hours of receiving the payment.

Privacy Policy
Our website adheres to the guidelines given under the General Data Protection Regulation (GDPR). This privacy policy aims to assure the safety of data for users visiting our website.

Transactions on this website are protected by up to 256-bit Secure Sockets Layer encryption.

Report Customization
We provide report customization options, wherein the buyer can structure the report according to their requirements.

24/7 Research Support
All queries are resolved from an industry expert.
Free Sample PDF Copy
Our Report will provide insights on Market size, Company Details and Total Addressable Market(TAM)
New Markets | New Geographies | Competition
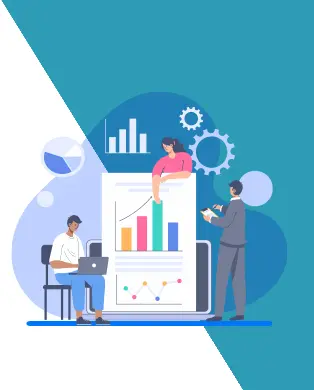
- Open access
- Published: 27 March 2024
Complex interplay of neurodevelopmental disorders (NDDs), fractures, and osteoporosis: a mendelian randomization study
- Zefang Li 1 ,
- Xueqiang Wu 2 ,
- Hanzheng Li 1 ,
- Cong Bi 3 ,
- Can Zhang 4 ,
- Yiqing Sun 1 &
- Zhaojun Yan 5
BMC Psychiatry volume 24 , Article number: 232 ( 2024 ) Cite this article
141 Accesses
1 Altmetric
Metrics details
Neurodevelopmental disorders (NDDs), such as Attention-Deficit/Hyperactivity Disorder (ADHD), Autism Spectrum Disorder (ASD), and Tourette Syndrome (TS), have been extensively studied for their multifaceted impacts on social and emotional well-being. Recently, there has been growing interest in their potential relationship with fracture risks in adulthood. This study aims to explore the associations between these disorders and fracture rates, in order to facilitate better prevention and treatment.
Employing a novel approach, this study utilized Mendelian randomization (MR) analysis to investigate the complex interplay between ADHD, ASD, TS, and fractures. The MR framework, leveraging extensive genomic datasets, facilitated a systematic examination of potential causal relationships and genetic predispositions.
The findings unveil intriguing bidirectional causal links between ADHD, ASD, and specific types of fractures. Notably, ADHD is identified as a risk factor for fractures, with pronounced associations in various anatomical regions, including the skull, trunk, and lower limbs. Conversely, individuals with specific fractures, notably those affecting the femur and lumbar spine, exhibit an increased genetic predisposition to ADHD and ASD. In this research, no correlation was found between TS and fractures, or osteoporosis.These results provide a genetic perspective on the complex relationships between NDDs and fractures, emphasizing the importance of early diagnosis, intervention, and a holistic approach to healthcare.
This research sheds new light on the intricate connections between NDDs and fractures, offering valuable insights into potential risk factors and causal links. The bidirectional causal relationships between ADHD, ASD, and specific fractures highlight the need for comprehensive clinical approaches that consider both NDDs and physical well-being.
Peer Review reports
Introduction
Attention Deficit Hyperactivity Disorder (ADHD), Autism Spectrum Disorder (ASD), and Tourette Syndrome (TS) are prominent Neurodevelopmental disorders(NDDs)often co-occurring and impactingsocial and emotional aspects [ 1 ]. Observational studies reveal higher fracture risks among adults with these conditions compared to those without [ 2 ]. Notably, ADHD is associated with an increased risk of fractures, highlighting the importance of recognizing the elevated risk in individuals with ADHD [ 3 , 4 , 5 , 6 ].
The core features of ADHD involve attention deficits, heightened hyperactivity, and impulsive behaviors. Notably, children with ADHD face an elevated risk of fractures, particularly affecting the skull, neck, trunk, and intracranial structures, with a Hazard Ratio (HR) 3.07 times higher than neurotypical peers [ 7 ]. Research highlights the statistical significance ( P < 0.001) of this heightened fracture risk, encompassing regions such as the skull, neck, and trunk (HR = 1.53), upper limbs (HR = 1.28), and lower extremities (HR = 1.84) [ 5 ]. Moreover, studies indicate a correlation between ADHD symptoms and limb fractures in adults [ 8 ], with a notable reduction in fracture risk for individuals receiving pharmacological interventions [ 9 , 10 ].
ASD is a NDDs by social impairment and restricted interactive and communicative behaviors [ 11 ]. Notably, individuals within the ASD spectrum exhibit an increased susceptibility to injuries involving the head, face, and neck [ 12 ], as well as a heightened risk of fractures affecting the hip, forearm, and spine [ 13 ]. Additionally, adolescent boys with ASD display lower bone mineral density (BMD) in comparison to their neurotypical peers [ 14 ].
Research consistently reveals distinctive patterns in bone health among individuals with ASD, including reduced systemic bone mineral content, diminished cortical area, and thinning of cortical and trabecular bone structures, especially in the distal radius and tibia. These trends extend to various anatomical regions, such as the lumbar spine, hips, and femoral neck, setting individuals with ASD apart from those without the condition [ 15 ].
TS is an intricate NDDs characterized by the presence of persistent vocal and motor tics, a defining feature that must endure for at least one year. Notably, a comprehensive decade-long cohort study unveiled a 1.27-fold higher fracture rate in individuals within the TS cohort when compared to a meticulously matched control cohort. Furthermore, a thorough multivariate Cox regression analysis revealed an adjusted HR of 1.28. Of particular interest is the observation that the introduction of antipsychotic medications led to a notable reduction in this heightened risk, resulting in a decreased HR of 1.17 [ 16 ]. This phenomenon warrants investigation, considering factors such as self-harm, accidental injuries, and the potential consequences of antipsychotic medication-related side effects [ 16 , 17 ].
Fractures and osteoporosis cast a significant shadow over public health [ 18 ], with a disquieting projection of over 30 million individuals expected to be affected by bone-related conditions in Europe by the year 2050. The associated financial burden is equally disconcerting, with potential hospitalization costs in Europe alone estimated to reach up to €3.5 billion annually [ 19 ]. It is crucial to acknowledge that while genetics play a substantial role, one’s bone structure is subject to modification, influenced by intrinsic and extrinsic factors such as physical activity, hormonal dynamics, and nutritional status [ 20 ].
The pivotal role of nutrition and physical activity takes center stage in strengthening bones during crucial early developmental stages. This emphasis on early intervention stems from the understanding that as individuals progress through life, the gradual accrual of undesirable bone mass can lead to bone fragility and, ultimately, the onset of osteoporosis. Aligned with the insights of the International Osteoporosis Foundation, osteoporosis is aptly defined as a condition characterized by low bone mass and the progressive deterioration of bone microstructure, culminating in heightened bone fragility and an elevated risk of fractures [ 21 ].
Fractures in the Pediatric and Adolescent Realm: A Significant Component of Injuries:
Fractures hold a significant place in the realm of accidents and injuries, accounting for a substantial 25% of all such incidents involving children and adolescents. While a wide array of risk factors has been identified, including factors like nutritional deficiencies [ 22 ], high body weight, and intense physical activities [ 23 ], as well as psychiatric disorders such as schizophrenia [ 24 ], the intricate interplay between fractures, osteoporosis, and the three NDDs of ADHD, ASD, and TS warrants comprehensive examination.
Simultaneously, cross-disease investigations have uncovered significant overlap in the phenotypic traits associated with the genetic risk shared by ADHD, ASD, and TS [ 1 ]. Grounded in the characteristic features of inattention, hyperactivity, and impulsivity commonly observed in individuals with ADHD, previous research provides a compelling basis for postulating causal links between ADHD, ASD, TS and the occurrence of fractures and osteoporosis. Furthermore, considering the associations among ADHD, ASD, TS, and intellectual disabilities, all of which fall within the spectrum of NDDs [ 25 , 26 ], we have deliberately incorporated intellectual functioning into the scope of our investigation to enhance the credibility of our research. It is essential to recognize that these causal relationships may exhibit distinct patterns across various anatomical sites.
Mendelian randomization (MR) methodologies harness the potential of single nucleotide polymorphisms (SNPs) as instrumental variables(IVs) to rigorously estimate the causal relationships between exposures and specific outcomes. This approach offers a distinct advantage by significantly mitigating the impact of confounding variables and measurement errors that often confound traditional multivariate regression models [ 27 ]. Moreover, the deliberate exclusion of IVs that overlap with confounding factors in MR analyses minimizes the risk of bias, thereby enhancing the validity of causal inferences [ 28 ].
Our primary aim is to utilize the robust MR methodology for a comprehensive evaluation of the causal associations between ADHD, ASD, TS and an elevated genetic predisposition to fractures in diverse anatomical regions.
Data source for NDDs
The genomic association data for ADHD [ 29 ], ASD [ 30 ], TS [ 31 ] utilized in this study were extracted from the PGC database. The genomic association data for intelligence [ 32 ] were extracted from Complex Trait Genetics Lab (CTG) ( https://ctg.cncr.nl/ ).
The Population Characteristics:
All participants included in the study were of European descent. The diagnostic criteria for ADHD and ASD were based on the ICD-10 (International Classification of Diseases, 10th Revision), while the diagnostic criteria for Tourette Disorder adhered to the DSM-5 (Diagnostic and Statistical Manual of Mental Disorders, Fifth Edition).
Instrumental Variables (IVs):
The IVs employed in the analysis consisted of a total of 582 SNPs. These SNPs were meticulously selected based on specific criteria, including a significance threshold of p < 5 × 10^-6, minimal linkage disequilibrium (LD) interlocking imbalance (LD r^2 < 0.001), and a clumping distance exceeding 10,000 kilobases. Detailed information about these IVs can be found in Supplementary Table.
Data source for fractures and osteoporosis
The data related to fractures and osteoporosis in this study were sourced from the FinnGen project [ 33 ], specifically utilizing the R9 version of the data, which was published on May 11, 2023. Detailed instructions and information about this research project and its data sources are available on the project’s website: FinnGen Project Information.
Diagnostic Criteria and Participant Characteristics:
The diagnosis of fractures and osteoporosis across these categories was based on the ICD-10. All individuals included in the study were of European descent.
IVs comprised a total of 269 SNPs. These SNPs were carefully selected based on specific criteria, including a significance threshold of p < 5 × 10^-6, minimal LD interlocking imbalance (LD r^2 < 0.001), and a clumping distance exceeding 10,000 kilobases. Comprehensive information regarding these IVs can be found in Supplementary Table.
The Details of cohort specifics of NDDs and Fractures and Osteoporosis are presented in Table 1 .
Confounding factors
Numerous studies have highlighted a significant connection between early life-related traits and the emergence of neurological and psychiatric disorders in adulthood, potentially due to increased oxidative stress within the central nervous system during early life [ 34 ]. For example, a well-documented association exists between low birth weight and ADHD [ 35 ]. Additionally, the mounting prevalence of childhood obesity has emerged as a significant public health concern due to its intricate and multifaceted relationship with neurodevelopmental health challenges in adulthood [ 36 ]. Furthermore, it is worth mentioning that allergic diseases during childhood hold the potential to precipitate ADHD symptoms [ 37 ].
Management of Confounding Factors:
Moreover, existing literature has indicated that the intake of vitamin D [ 38 ], calcium [ 39 ], and zinc [ 40 ] serve as risk factors for fractures and osteoporosis. Supplementation with vitamin D has been shown to augment bone density, thereby mitigating the risks of fractures and osteoporosis [ 38 ]. Calcium and vitamin D have been identified as pivotal determinants of peak bone mass [ 39 ]. Conversely, a diminished intake of zinc is associated with an elevated risk of fractures and osteoporosis [ 40 ]. Consequently, we have incorporated dietary supplements of vitamin D, calcium, and zinc as confounding variables in this study.
In light of the aforementioned considerations, this study rigorously controlled for potential confounding factors. It incorporated birth height [ 41 ], birth weight [ 42 ], body mass index (BMI) [ 43 ], and the presence of allergic diseases within the analytical framework. The impact of these factors on the observed outcomes was meticulously addressed by excluding SNPs that showed overlap within the IVs. Birth height, weight, and children’s BMI data were sourced from The Early Growth Genetics (EGG) Consortium, while information on allergic diseases was acquired from a Finnish database [ 33 ]. The data on theintakeof vitamin D, calcium, and zinc were sourced from the IEU Open GWAS database [ 44 ]. This comprehensive approach enhances the study’s robustness, minimizing the influence of potential confounders and bolstering the precision of causal inferences.
Statistical analysis
This study employed a MR approach to explore the intricate bidirectional relationship between ADHD, ASD, TS, and 11 distinct types of fractures and osteoporosis.
The study adhered to the classical MR assumptions (Fig. 1 )
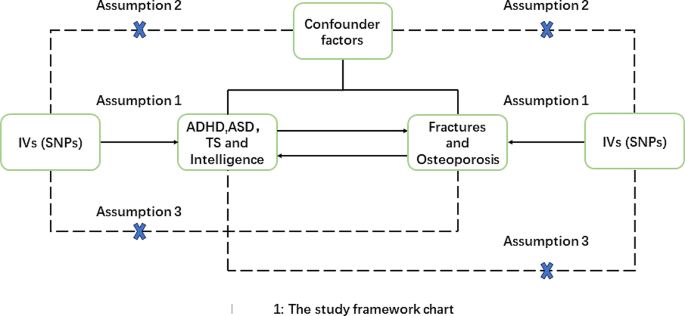
MR assumptions and design of our study:1. IV Exposure Association: IVs demonstrated a robust association with the exposure of interest.2. Absence of Unidentified Confounding: IVs were selected to have no associations with unidentified confounding factors that could affect both the exposure and outcome.3. IV Effect via Exposure: IVs exclusively influenced the outcomes through the exposure under investigation
To mitigate confounding factors, the study rigorously screened IVs and established a significance threshold ( P < 5e-06) to minimize weak associations between potential confounders and genetic variations.
The primary statistical metric used was the inverse variance weighted (IVW) method, assessing the association between ADHD, ASD, and fractures or osteoporosis. MR-Egger, weighted median, and weighted models served as supplementary statistical indicators.
Sensitivity analysis, a crucial component of MR analysis, was employed to detect potential pleiotropy. Heterogeneity was assessed using the Cochrane Q test, and horizontal pleiotropy was examined via the MR-Egger intercept method. A leave-one-out analysis assessed the impact of individual SNPs on MR results. In cases of heterogeneity, the random-effect IVW was used as the primary statistical measure, while the fixed-effect IVW was employed when no heterogeneity was observed.
The significance threshold for results was corrected for multiple testing using the Bonferroni method. A p-value of < 0.001 (0.05/36) was considered significant, p-values between 0.001 and 0.05 were considered suggestive, and p-values above 0.05 were deemed insignificant. The F statistic \( (F=\frac{bet{a}^{2}}{s{e}^{2}})\) was used to assess the strength of IVs, with F > 10 indicating a robust and independent instrumental variable [ 45 ].
All statistical analyses were performed using the TwoSample MR package in R version 4.2.2, and the results were reported in terms of odds ratio (OR) values with associated 95% confidence intervals.
Causal relationship between fractures and osteoporosis and ADHD, ASD, TS and intelligence
A similar screening process, accounting for P-values and LD clumping, was applied to SNPs used as IVs for different fracture and osteoporosis outcomes. Although potential causal relationships were detected, they did not consistently reach statistical significance after Bonferroni correction (Fig. 2 )
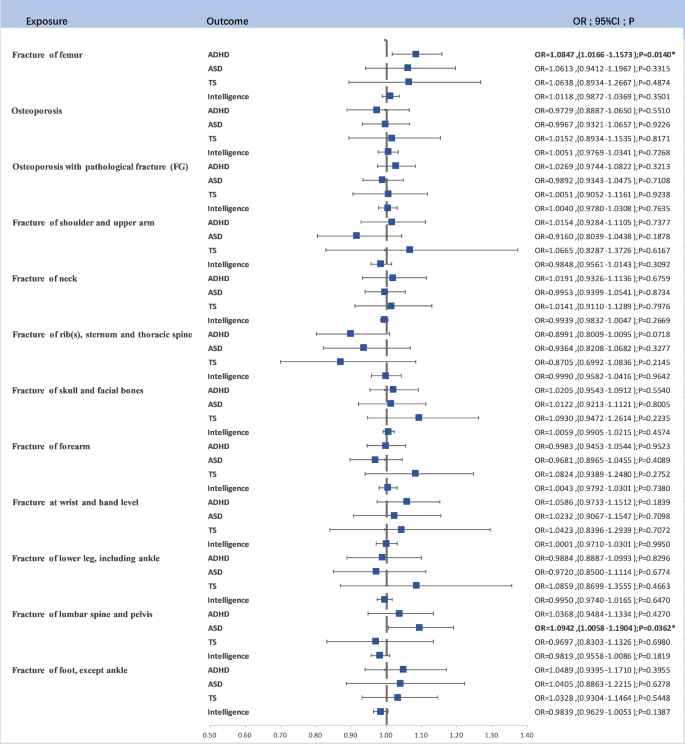
The forest map for Causal Relationship Between Fractures and Osteoporosis and ADHD, ASD, and TS;*: 0.05 < P < 0.001, *** P < 0.001
Fracture of femur and ADHD: A suggestive causal relationship was observed (OR = 1.0847, 95% CI (1.0166–1.1573), P = 0.0140).
Fracture of lumbar spine and pelvis and ASD: Another suggestive causal relationship was noted (OR = 1.0942, 95% CI (1.0058–1.1904), P = 0.0362).
These findings, while suggestive, did not maintain statistical significance following Bonferroni correction but offer valuable insights into potential risk factors.
Heterogeneity and Pleiotropy Testing:
Detailed information on the results of heterogeneity and pleiotropy testing is available in the supplementary material and supplementary table, providing a comprehensive assessment of the findings’ robustness in light of these factors.
Causal relationship between ADHD, ASD, TS, intelligence and fracture risk
After rigorous SNP screening based on P-values and LD clumping, a total of 138, 34, 22 and 387 linkage-disequilibrium-independent SNPs served as IVs for ADHD, ASD, TS and Intelligence. Upon eliminating SNPs not present in the outcome variable, a two-sample MR analysis unveiled significant findings (Fig. 3 ).
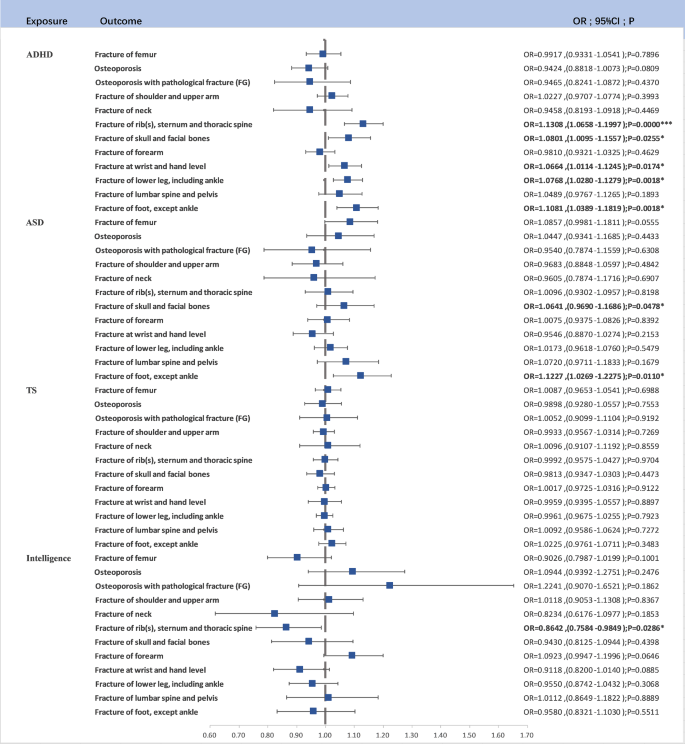
The forest map for Causal Relationship Between ADHD, ASD, TS, and Fracture Risk*: 0.05 < P < 0.001, *** P < 0.001
ADHD as a Risk Factor: ADHD was identified as a risk factor for Fracture of rib(s), sternum, and thoracic spine (OR = 1.1308, 95% CI (1.0658–1.1997), P < 0.0001). This association retained significance after Bonferroni correction. ADHD was also linked to Fracture of foot (except ankle) (OR = 1.1081, 95% CI (1.0389–1.1819), P = 0.0018), Fracture of lower leg (including ankle) (OR = 1.0768, 95% CI (1.0280–1.1279), P = 0.0018), Fracture at wrist and hand level (OR = 1.0664, 95% CI (1.0114–1.1245), P = 0.0174), and Fracture of skull and facial bones (OR = 1.0801, 95% CI (1.0095–1.1557), P = 0.0255).
ASD as a Risk Factor: ASD was found to be associated with an increased risk of Fracture of foot (except ankle) (OR = 1.1227, 95% CI (1.0269–1.2275), P = 0.0110).
TS as a Risk Factor: No significant causal relationship wasfoundin TS.
Additionally, we observed that higher levels of intelligence were associated with a reduced risk of fractures involving the rib(s), sternum, and thoracic spine (OR = 0.8642, 95% CI (0.7584–0.9849), P = 0.0286). The pleiotropy and heterogeneity test results are included in the supplementary materials.
This comprehensive study adopted a bidirectional MR approach to investigate the intricate relationships between three NDDs—ADHD, ASD, and TS—and a spectrum of 11 distinct fractures and osteoporosis. The MR analysis provided intriguing insights into these associations, shedding light on potential causal links and risk factors (Fig. 4 ).
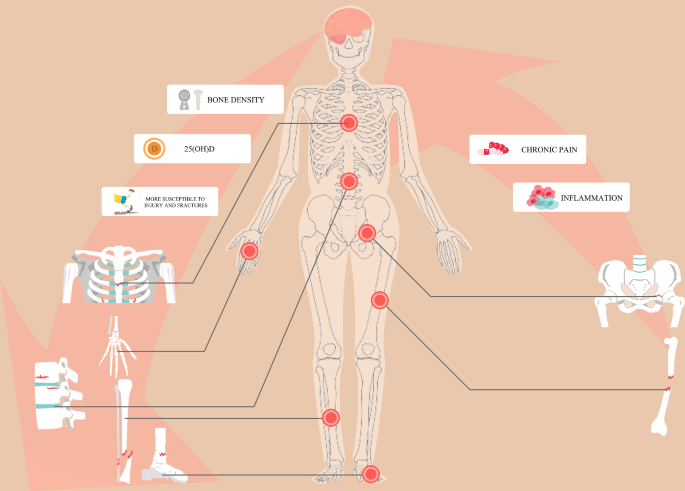
The Potential mechanisms for our study
Fractures as causal factors
The MR analysis results suggested that certain fractures may act as causal factors in the development of these childhood NDDs. Specifically, a fracture of the femur may be a risk factor for ADHD ( P = 0.0140), and fractures of the lumbar spine and pelvis may be risk factors for ASD ( P = 0.0362). Although these findings did not retain statistical significance after Bonferroni correction, they offer valuable indications of potential associations.
NDDs as causal factors
Conversely, the reverse MR analysis presented robust findings indicating that ADHD could be a causal factor for various types of fractures. For example, ADHD is a risk factor for fractures of the rib(s), sternum, and thoracic spine ( P < 0.0001), fractures of the foot (except ankle) ( P = 0.0018), fractures of the lower leg (including ankle) ( P = 0.0003), fractures at the wrist and hand level ( P = 0.0174), and fractures of the craniofacial region ( P = 0.0255). Similarly, ASD is considered a risk factor for foot fractures ( P = 0.0110), and higher intelligence levels are associated with a preventive effect against the occurrence of fractures involving the rib(s), sternum, and thoracic spine ( P = 0.0286). Besides, there is no causal relationship between fractures and TS.
Observational studies have historically grappled with the intricate connections among ADHD, ASD, TS, and fractures, as well as osteoporosis. Often, symptoms linked to these NDDs may unintentionally escape attention when investigating fractures [ 46 ]. Our study represents a pioneering effort, constituting the inaugural systematic exploration of the link between childhood NDDs and fractures and osteoporosis through a genetic framework. By adeptly sidestepping potential confounding variables, measurement inaccuracies, and other sources of bias, the study yields invaluable genetic insights. Significantly, it identifies NDDs like ADHD as potential contributors to various fractures, while also suggesting that fractures might be potential causes of diverse fractures. However, this perspective requires further research for clarification. It introduces a new perspective on the potential interplay between psychological well-being and bone health.
Fractures not only induce pain and inflammatory reactions but recent research has also uncovered connections between NDDs and pain [ 47 , 48 ]. Interestingly, the prevalence of ADHD is significantly higher in adolescents experiencing chronic pain [ 49 , 50 , 51 ], and inflammation is suggested as a contributing factor in the development of ADHD and ASD [ 52 , 53 ]. For instance, inflammatory pain in newborns and responses in preterm infants may elevate the risk of NDDs [ 54 ]. In individuals with ADHD, elevated levels of inflammatory biomarkers and inflammation within the central nervous system are believed to contribute to pain perception and sensitization [ 54 , 55 ]. This also holds promise for preventing chronic pain in adults with ADHD through neuroinflammation treatment [ 56 ].
Shifting focus to the post-traumatic inflammatory response, microglia and their associated molecular pathways play a pivotal role [ 57 ], especially in scenarios like traumatic brain injury (TBI) [ 58 ]. Research indicates that TBI coincides with heightened neuroinflammatory responses, as observed in mice with tibial fractures [ 59 ]. These responses are concurrent with disruptions in the blood-brain barrier and behavioral deficits. The increase in interleukin-1β (IL-1β) levels in the brain is associated with these changes, influencing astrocytes, microglia, and neurons while governing neuroinflammatory processes [ 57 , 60 ]. This series of findings underscores the intricate interplay among fractures, NDDs, inflammation, and pain.
Vitamin D is crucial for both musculoskeletal well-being and brain function. It is indispensable for maintaining healthy bones, but its deficiency can elevate the risk of fractures [ 61 , 62 ]. In terms of brain function, vitamin D functions as a neurosteroid hormone, influencing neurodevelopment processes such as nerve cell proliferation, neurotransmission, oxidative stress regulation, and immune function—all of which are integral to the central nervous system [ 63 , 64 ].
Vitamin D deficiency has been linked to various NDDs and psychiatric disorders, including ADHD, ASD, and schizophrenia [ 65 , 66 , 67 ]. Individuals with ADHD and ASD frequently exhibit lower levels of 25-hydroxyvitamin D (25(OH)D) in their serum compared to their healthy counterparts [ 68 , 69 ]. Moreover, an inverse relationship exists between vitamin D and its receptor levels (VDR) and the severity of ASD and ADHD [ 70 , 71 ]. This suggests that vitamin D may play a significant role not only in the development of ASD but also in ADHD and fractures.
In the context of ASD, children typically engage in less physical activity, have a lower BMI, and may not consume adequate amounts of calcium and calories [ 72 , 73 ]. These factors collectively increase the risk of low bone density in individuals with ASD [ 15 , 74 ]. For ADHD, various factors contribute to injuries and fractures in affected children. Some of these incidents can be attributed to the unique characteristics of ADHD [ 75 ]. Impulsivity is a hallmark symptom of ADHD, characterized by actions taken without prior thought, insufficient judgment, and a tendency to seek immediate gratification [ 10 , 75 ]. Research suggests that children with ADHD, despite being capable of recognizing dangers, tend to underestimate the severity of consequences arising from their behaviors [ 76 ]. This inherent impulsivity may render children with ADHD more susceptible to injuries and fractures, even in situations comparable to their peers without ADHD [ 75 ]. Similarly, low intelligence in the early years has been shown to increase the risk of unintentional injury [ 77 ].
However, it is crucial to acknowledge certain limitations in our study. Firstly, we did not analyze the causal relationship between ADHD, ASD, and fractures based on variables such as sex, age, BMI, and other relevant factors. Nevertheless, existing research suggests that these factors could potentially influence causation. Secondly, our study exclusively relied on genetic data from individuals of European ancestry, and we did not validate our findings in non-European populations. As a result, the generalizability of our conclusions to non-European populations remains uncertain.
While our study has several important strengths, providing insights into healthcare management strategies for children with ADHD, it is essential to recognize its limitations. Our findings emphasize the importance of prioritizing the bone health of children with ADHD by monitoring their BMD and implementing appropriate vitamin D supplementation strategies. Furthermore, our results may prompt a shift in the approach to fractures in children. ADHD symptoms are often overlooked in the context of fracture management, and our study aims to address this gap. One key strength of our research lies in the application of the MR method. This approach effectively addresses the potential influence of external social factors on the relationship between exposure variables and outcomes, thereby enhancing the robustness and reliability of our findings.
Data availability
Summary data on ADHD, ASD, TS, Fractures and Osteoporosis is available on supplementary material.
Jain P, Miller-Fleming T, Topaloudi A, Yu D, Drineas P, Georgitsi M, Yang Z, Rizzo R, Müller-Vahl KR, Tumer Z et al. Polygenic risk score-based phenome-wide association study identifies novel associations for Tourette syndrome. Translational Psychiatry 2023, 13(1).
Whitney DG, Caird MS, Jepsen KJ, Kamdar NS, Marsack-Topolewski CN, Hurvitz EA, Peterson MD. Elevated fracture risk for adults with neurodevelopmental disabilities. Bone 2020, 130.
Chou IC, Lin CC, Sung FC, Kao CH. Attention-deficit‐hyperactivity disorder increases risk of bone fracture: a population‐based cohort study. Dev Med Child Neurol. 2014;56(11):1111–6.
Article PubMed Google Scholar
Perry BA, Archer KR, Song Y, Ma Y, Green JK, Elefteriou F, Dahir KM. Medication therapy for attention deficit/hyperactivity disorder is associated with lower risk of fracture: a retrospective cohort study. Osteoporos Int. 2016;27(7):2223–7.
Article CAS PubMed Google Scholar
Guo N-W, Lin C-L, Lin C-W, Huang M-T, Chang W-L, Lu T-H, Lin C-J. Fracture risk and correlating factors of a pediatric population with attention deficit hyperactivity disorder: a nationwide matched study. J Pediatr Orthop B. 2016;25(4):369–74.
Prasad V, West J, Sayal K, Kendrick D. Injury among children and young people with and without attention-deficit hyperactivity disorder in the community: the risk of fractures, thermal injuries, and poisonings. Child Care Health Dev. 2018;44(6):871–8.
Merrill RM, Lyon JL, Baker RK, Gren LH. Attention deficit hyperactivity disorder and increased risk of injury. Adv Med Sci. 2009;54(1):20–6.
Kömürcü E, Bilgiç A, Hergüner S. Relationship between extremity fractures and attention-deficit/hyperactivity disorder symptomatology in adults. Int J Psychiatry Med. 2014;47(1):55–63.
Jacob L, Kostev K. Impact of attention deficit hyperactivity disorder therapy on fracture risk in children treated in German pediatric practices. Osteoporos Int. 2017;28(4):1265–9.
Ziv-Baran T, Zacay G, Modan-Moses D, Reingold SM, Mekori E, Levy-Shraga Y. Increased fracture risk among children diagnosed with attention- deficit/hyperactivity disorder: a large matched cohort study. Eur J Pediatrics. 2023;182(6):2705–14.
Article Google Scholar
Hashem S, Nisar S, Bhat AA, Yadav SK, Azeem MW, Bagga P, Fakhro K, Reddy R, Frenneaux MP, Haris M. Genetics of structural and functional brain changes in autism spectrum disorder. Translational Psychiatry 2020, 10(1).
McDermott S, Zhou L, Mann J. Injury Treatment among children with autism or pervasive developmental disorder. J Autism Dev Disord. 2007;38(4):626–33.
Neumeyer AM, O’Rourke JA, Massa A, Lee H, Lawson EA, McDougle CJ, Misra M. Brief report: bone fractures in children and adults with Autism Spectrum disorders. J Autism Dev Disord. 2014;45(3):881–7.
Ekhlaspour L, Baskaran C, Campoverde KJ, Sokoloff NC, Neumeyer AM, Misra M. Bone density in adolescents and young adults with Autism Spectrum disorders. J Autism Dev Disord. 2016;46(11):3387–91.
Article PubMed PubMed Central Google Scholar
Rostami Haji Abadi M, Neumeyer A, Misra M, Kontulainen S. Bone health in children and youth with ASD: a systematic review and meta-analysis. Osteoporos Int. 2021;32(9):1679–91.
Lu Y-Y, Wang M-Y, Wei IH, Lin C-C, Huang C-C. Tourette syndrome increases risk of bone fractures: a population-based cohort study. Eur Child Adolesc Psychiatry. 2016;26(5):531–9.
Armenteros JL, Davies M. Antipsychotics in early onset Schizophrenia. Eur Child Adolesc Psychiatry. 2006;15(3):141–8.
Kanis JA. Diagnosis of osteoporosis and assessment of fracture risk. Lancet. 2002;359(9321):1929–36.
Wilson-Barnes SL, Lanham-New SA, Lambert H. Modifiable risk factors for bone health & fragility fractures. Best Pract Res Clin Rheumatol 2022, 36(3).
Boudin E, Fijalkowski I, Hendrickx G, Van Hul W. Genetic control of bone mass. Mol Cell Endocrinol. 2016;432:3–13.
Cosman F, de Beur SJ, LeBoff MS, Lewiecki EM, Tanner B, Randall S, Lindsay R. Clinician’s guide to Prevention and treatment of osteoporosis. Osteoporos Int. 2014;25(10):2359–81.
Article CAS PubMed PubMed Central Google Scholar
Denova-Gutiérrez E, Méndez-Sánchez L, Muñoz-Aguirre P, Tucker KL, Clark P. Dietary patterns, bone Mineral Density, and risk of fractures: a systematic review and Meta-analysis. Nutrients 2018, 10(12).
Lane JC, Butler KL, Poveda-Marina JL, Martinez-Laguna D, Reyes C, de Bont J, Javaid MK, Logue J, Compston JE, Cooper C, et al. Preschool obesity is Associated with an increased risk of Childhood fracture: a longitudinal cohort study of 466,997 children and up to 11years of follow-up in Catalonia, Spain. J Bone Min Res. 2020;35(6):1022–30.
Jia N, Dong L, Lu Q, Li X, Jin M, Yin X, Zhu Z, Jia Q, Ji C, Hui L, et al. The causal effect of schizophrenia on fractures and bone mineral density: a comprehensive two-sample mendelian randomization study of European ancestry. BMC Psychiatry. 2023;23(1):692.
Diagnostic and statistical manual of mental disorders: DSM-5™, 5th ed. In: Diagnostic and statistical manual of mental disorders: DSM-5™, 5th ed. Arlington, VA, US: American Psychiatric Publishing, Inc. 2013: xliv, 947-xliv, 947.
Åsberg Johnels J, Yngvesson P, Billstedt E, Gillberg C, Halldner L, Råstam M, Gustafsson P, Selinus EN, Lichtenstein P, Hellner C, et al. The relationship between intelligence and global adaptive functioning in young people with or without neurodevelopmental disorders. Psychiatry Res. 2021;303:114076.
Davey Smith G, Hemani G. Mendelian randomization: genetic anchors for causal inference in epidemiological studies. Hum Mol Genet. 2014;23(R1):R89–98.
Richmond RC, Anderson EL, Dashti HS, Jones SE, Lane JM, Strand LB, Brumpton B, Rutter MK, Wood AR, Straif K et al. Investigating causal relations between sleep traits and risk of breast cancer in women: mendelian randomisation study. BMJ 2019.
Demontis D, Walters GB, Athanasiadis G, Walters R, Therrien K, Nielsen TT, Farajzadeh L, Voloudakis G, Bendl J, Zeng B, et al. Genome-wide analyses of ADHD identify 27 risk loci, refine the genetic architecture and implicate several cognitive domains. Nat Genet. 2023;55(2):198–208.
Grove J, Ripke S, Als TD, Mattheisen M, Walters RK, Won H, Pallesen J, Agerbo E, Andreassen OA, Anney R, et al. Identification of common genetic risk variants for autism spectrum disorder. Nat Genet. 2019;51(3):431–44.
Yu D, Sul JH, Tsetsos F, Nawaz MS, Huang AY, Zelaya I, Illmann C, Osiecki L, Darrow SM, Hirschtritt ME, et al. Interrogating the genetic determinants of Tourette’s syndrome and other Tic disorders through genome-wide Association studies. Am J Psychiatry. 2019;176(3):217–27.
Savage JE, Jansen PR, Stringer S, Watanabe K, Bryois J, de Leeuw CA, Nagel M, Awasthi S, Barr PB, Coleman JRI, et al. Genome-wide association meta-analysis in 269,867 individuals identifies new genetic and functional links to intelligence. Nat Genet. 2018;50(7):912–9.
Kurki MI, Karjalainen J, Palta P, Sipilä TP, Kristiansson K, Donner KM, Reeve MP, Laivuori H, Aavikko M, Kaunisto MA, et al. FinnGen provides genetic insights from a well-phenotyped isolated population. Nature. 2023;613(7944):508–18.
Schiavone S, Colaianna M, Curtis L. Impact of early life stress on the Pathogenesis of Mental disorders: relation to Brain oxidative stress. Curr Pharm Design. 2015;21(11):1404–12.
Article CAS Google Scholar
Momany AM, Kamradt JM, Nikolas MA. A Meta-analysis of the Association between Birth Weight and attention deficit hyperactivity disorder. J Abnorm Child Psychol. 2018;46(7):1409–26.
He R, Mo J, Zhu K, Luo Q, Liu X, Huang H, Sheng J. The early life course-related traits with three psychiatric disorders: a two-sample mendelian randomization study. Front Psychiatry 2023, 14.
Xu X, Zhuo L, Zhang L, Peng H, Lyu Y, Sun H, Zhai Y, Luo D, Wang X, Li X, et al. Dexmedetomidine alleviates host ADHD-like behaviors by reshaping the gut microbiota and reducing gut-brain inflammation. Psychiatry Res. 2023;323:115172.
Lips P, van Schoor NM. The effect of vitamin D on bone and osteoporosis. Best Pract Res Clin Endocrinol Metab. 2011;25(4):585–91.
Vannucci L, Masi L, Gronchi G, Fossi C, Carossino AM, Brandi ML. Calcium intake, bone mineral density, and fragility fractures: evidence from an Italian outpatient population. Archives Osteoporos 2017, 12(1).
Ceylan MN, Akdas S, Yazihan N. Is zinc an important Trace element on bone-related diseases and complications? A Meta-analysis and systematic review from serum level, Dietary Intake, and supplementation aspects. Biol Trace Elem Res. 2020;199(2):535–49.
van der Valk RJP, Kreiner-Møller E, Kooijman MN, Guxens M, Stergiakouli E, Sääf A, Bradfield JP, Geller F, Hayes MG, Cousminer DL, et al. A novel common variant in DCST2 is associated with length in early life and height in adulthood. Hum Mol Genet. 2015;24(4):1155–68.
Warrington NM, Beaumont RN, Horikoshi M, Day FR, Helgeland Ø, Laurin C, Bacelis J, Peng S, Hao K, Feenstra B, et al. Maternal and fetal genetic effects on birth weight and their relevance to cardio-metabolic risk factors. Nat Genet. 2019;51(5):804–14.
Copenhaver GP, Vogelezang S, Bradfield JP, Ahluwalia TS, Curtin JA, Lakka TA, Grarup N, Scholz M, van der Most PJ, Monnereau C et al. Novel loci for childhood body mass index and shared heritability with adult cardiometabolic traits. PLoS Genet 2020, 16(10).
Elsworth B, Lyon M, Alexander T, Liu Y, Matthews P, Hallett J, Bates P, Palmer T, Haberland V, Smith GD et al. The MRC IEU OpenGWAS data infrastructure. 2020:2020.2008.2010.244293.
Yao C, Zhang Y, Lu P, Xiao B, Sun P, Tao J, Cheng Y, Kong L, Xu D, Fang M. Exploring the bidirectional relationship between pain and mental disorders: a comprehensive mendelian randomization study. J Headache Pain 2023, 24(1).
Davison R, Daniel JA, Idarraga AJ, Perticone KM, Lin J, Holmes GB, Lee S, Hamid KS, Bohl DD. Depression following operative treatments for Achilles ruptures and Ankle fractures. Foot Ankle Int. 2021;42(12):1579–83.
Low Kapalu CM, Hall JJ, Wallace DP. Neuropsychological functioning of Youth receiving Intensive Interdisciplinary Pain Treatment. J Pediatr Psychol. 2018;43(8):870–81.
Battison E, Brown P, Holley A, Wilson A. Associations between Chronic Pain and attention-deficit hyperactivity disorder (ADHD) in youth: a scoping review. Children 2023, 10(1).
Hechler T, Kanstrup M, Holley AL, Simons LE, Wicksell R, Hirschfeld G, Zernikow B. Systematic review on Intensive Interdisciplinary Pain Treatment of Children with Chronic Pain. Pediatrics. 2015;136(1):115–27.
Wiwe Lipsker C, von Heijne M, Bölte S, Wicksell RK. A case report and literature review of autism and attention deficit hyperactivity disorder in paediatric chronic pain. Acta Paediatr. 2018;107(5):753–8.
Wiwe Lipsker C, Bölte S, Hirvikoski T, Lekander M, Holmström L, Wicksell RK. Prevalence of autism traits and attention-deficit hyperactivity disorder symptoms in a clinical sample of children and adolescents with chronic pain. J Pain Res. 2018;11:2827–36.
Bryn V, Aass HC, Skjeldal OH, Isaksen J, Saugstad OD, Ormstad H. Cytokine Profile in Autism Spectrum disorders in Children. J Mol Neurosci. 2017;61(1):1–7.
Anand D, Colpo GD, Zeni G, Zeni CP, Teixeira AL. Attention-Deficit/Hyperactivity disorder and inflammation: what does current knowledge tell us? A systematic review. Front Psychiatry 2017, 8.
Lee JH, Espinera AR, Chen D, Choi K-E, Caslin AY, Won S, Pecoraro V, Xu G-Y, Wei L, Yu SP. Neonatal inflammatory pain and systemic inflammatory responses as possible environmental factors in the development of autism spectrum disorder of juvenile rats. J Neuroinflamm 2016, 13(1).
Koç S, Güler EM, Derin S, Gültekin F, Aktaş S. Oxidative and inflammatory parameters in children and adolescents with ADHD. J Atten Disord. 2023;27(8):880–6.
Kerekes N, Sanchéz-Pérez AM, Landry M. Neuroinflammation as a possible link between attention-deficit/hyperactivity disorder (ADHD) and pain. Med Hypotheses. 2021;157:110717.
Prata J, Santos SG, Almeida MI, Coelho R, Barbosa MA. Bridging Autism Spectrum disorders and Schizophrenia through inflammation and biomarkers - pre-clinical and clinical investigations. J Neuroinflamm 2017, 14(1).
Urrutia-Ruiz C, Rombach D, Cursano S, Gerlach-Arbeiter S, Schoen M, Bockmann J, Demestre M, Boeckers TM. Deletion of the Autism-Associated protein SHANK3 abolishes structural synaptic plasticity after Brain Trauma. Int J Mol Sci 2022, 23(11).
Lu S-M, Yu C-J, Liu Y-H, Dong H-Q, Zhang X, Zhang S-S, Hu L-Q, Zhang F, Qian Y-N, Gui B. S100A8 contributes to postoperative cognitive dysfunction in mice undergoing tibial fracture surgery by activating the TLR4/MyD88 pathway. Brain Behav Immun. 2015;44:221–34.
Pike AF, Varanita T, Herrebout MAC, Plug BC, Kole J, Musters RJP, Teunissen CE, Hoozemans JJM, Bubacco L, Veerhuis R. α-Synuclein evokes NLRP3 inflammasome‐mediated IL‐1β secretion from primary human microglia. Glia. 2021;69(6):1413–28.
Silva ICJ, Lazaretti-Castro M. Vitamin D metabolism and extraskeletal outcomes: an update. Archives Endocrinol Metabolism. 2022;66(5):748–55.
Dixon KM, Mason RS. Vitamin D. Int J Biochem Cell Biol. 2009;41(5):982–5.
Rihal V, khan H, Kaur A, Singh TG, Abdel-Daim MM. Therapeutic and mechanistic intervention of vitamin D in neuropsychiatric disorders. Psychiatry Res. 2022;317:114782.
Ali A, Cui X, Eyles D. Developmental vitamin D deficiency and autism: putative pathogenic mechanisms. J Steroid Biochem Mol Biol. 2018;175:108–18.
Ye X, Zhou Q, Ren P, Xiang W, Xiao L. The Synaptic and Circuit Functions of Vitamin D in Neurodevelopment disorders. Neuropsychiatr Dis Treat. 2023;19:1515–30.
Gáll Z, Székely O. Role of vitamin D in cognitive dysfunction: new molecular concepts and discrepancies between animal and human findings. Nutrients. 2021;13(11):3672.
Siracusano M, Riccioni A, Abate R, Benvenuto A, Curatolo P, Mazzone L. Vitamin D Deficiency and Autism Spectrum Disorder. Curr Pharm Design. 2020;26(21):2460–74.
Kotsi E, Kotsi E, Perrea DN. Vitamin D levels in children and adolescents with attention-deficit hyperactivity disorder (ADHD): a meta-analysis. ADHD Atten Deficit Hyperactivity Disorders. 2019;11(3):221–32.
Saedisomeolia A, Samadi M, Gholami F, Seyedi M, Effatpanah M, Hashemi R, Abdolahi M, Honarvar MN. Vitamin D’s molecular action mechanism in Attention-Deficit/ hyperactivity disorder: a review of evidence. CNS Neurol Disorders - Drug Targets. 2018;17(4):280–90.
Ogbu D, Xia E, Sun J. Gut instincts: vitamin D/vitamin D receptor and microbiome in neurodevelopment disorders. Open Biology 2020, 10(7).
Ganta A, Pillai SS, Fredette ME, Topor LS. Severe Vitamin D Deficiency in Youth with Autism Spectrum Disorder during the COVID-19 pandemic. J Dev Behav Pediatr. 2022;43(8):461–4.
Gehricke J-G, Chan J, Farmer JG, Fenning RM, Steinberg-Epstein R, Misra M, Parker RA, Neumeyer AM. Physical activity rates in children and adolescents with autism spectrum disorder compared to the general population. Res Autism Spectr Disorders 2020, 70.
Soden SE, Garrison CB, Egan AM, Beckwith AM. Nutrition, Physical Activity, and bone Mineral Density in Youth with Autistic Spectrum disorders. J Dev Behav Pediatr. 2012;33(8):618–24.
Calarge CA, Schlechte JA. Bone Mass in boys with Autism Spectrum Disorder. J Autism Dev Disord. 2017;47(6):1749–55.
Seens H, Modarresi S, MacDermid JC, Walton DM, Grewal R. Prevalence of bone fractures among children and adolescents with attention-deficit/hyperactivity disorder: a systematic review and meta-analysis. BMC Pediatr. 2021;21(1):354.
Farmer JE, Peterson L. Injury risk factors in children with attention deficit hyperactivity disorder. Health Psychol. 1995;14(4):325–32.
Whitley E, Batty GD, Gale CR, Deary IJ, Tynelius P, Rasmussen F. Intelligence in early adulthood and subsequent risk of unintentional injury over two decades: cohort study of 1 109 475 Swedish men. J Epidemiol Community Health. 2009;64(5):419–25.
Download references
Acknowledgements
We want to acknowledge the participants and investigatorsof the FinnGen, UK Biobank, Psychiatric GenomicsConsortium (PGC), The Early Growth Genetics(EGG) study
This research was supported by Jinan City’s “20 Colleges and Universities” funded project.
(No. 2020GXRC013); National Administration of Traditional Chinese Medicine Project(GZY-KJS-2023-017); Qingdao Science and Technology Benefiting the People Demonstration Special Project(23-7-8-smjk-3-nsh).
Author information
Authors and affiliations.
Department of The First Clinical medicine, Shandong University of Traditional Chinese Medicine, Jinan, China
Zefang Li, Hanzheng Li & Yiqing Sun
Department of Health Science, Shandong University of Traditional Chinese Medicine, Jinan, China
Xueqiang Wu
Department of Vascular Surgery, Shandong Provincial Hospital Affiliated to Shandong First Medical University, Jinan, China
School of Biomedical Sciences, Shandong First Medical University, Jinan, China
Affiliated Hospital of Shandong University of Traditional Chinese Medicine, Jinan, China
Zhaojun Yan
You can also search for this author in PubMed Google Scholar
Contributions
Xueqiang Wu and Zefang Li: conceptualization and writing-original draft. Hanzheng Li, Cong Bi, Can Zhang and Yiqing Sun: data curation and formal analysis. Zhaojun Yan funding acquisition and validation. Xueqiang Wu and Zhaojun Yan: methodology. All authors contributed to the article and approved the submitted version.
Corresponding authors
Correspondence to Xueqiang Wu or Zhaojun Yan .
Ethics declarations
Ethics approval and consent to participate.
Not applicable.
Consent for publication
Competing interests.
The authors declare no competing interests.
Additional information
Publisher’s note.
Springer Nature remains neutral with regard to jurisdictional claims in published maps and institutional affiliations.
Electronic supplementary material
Below is the link to the electronic supplementary material.
Supplementary Material 1:
Supplementary Material Supplementary Figure 1, 4, 7, 10 and 10-22 showed the leave one out plot of NDDs on bone fractures and osteoporosis; Figure 2, 5, 8 and 22 showed the funnel plot of NDDs on bone fractures and osteoporosis; Figure 3, 6, 9 and 23 showed the scatter plot of NDDs on bone fractures and osteoporosis. Supplementary Figure 24-26 showed the leave one out plot bone fractures and osteoporosis on NDDs; Supplementary Figure 27-29 showed the funnel plot bone fractures and osteoporosis on NDDs; Supplementary Figure 30-32 showed the scatter plot bone fractures and osteoporosis on NDDs
Supplementary Material 2:
Table S1, Summary data for exposure and outcome; Table S2, The IVs for our study; Table S3, The results for causal effect; Table S4, Results of heterogeneity test; Table S5, Results of sensitivity analysis
Rights and permissions
Open Access This article is licensed under a Creative Commons Attribution 4.0 International License, which permits use, sharing, adaptation, distribution and reproduction in any medium or format, as long as you give appropriate credit to the original author(s) and the source, provide a link to the Creative Commons licence, and indicate if changes were made. The images or other third party material in this article are included in the article’s Creative Commons licence, unless indicated otherwise in a credit line to the material. If material is not included in the article’s Creative Commons licence and your intended use is not permitted by statutory regulation or exceeds the permitted use, you will need to obtain permission directly from the copyright holder. To view a copy of this licence, visit http://creativecommons.org/licenses/by/4.0/ . The Creative Commons Public Domain Dedication waiver ( http://creativecommons.org/publicdomain/zero/1.0/ ) applies to the data made available in this article, unless otherwise stated in a credit line to the data.
Reprints and permissions
About this article
Cite this article.
Li, Z., Wu, X., Li, H. et al. Complex interplay of neurodevelopmental disorders (NDDs), fractures, and osteoporosis: a mendelian randomization study. BMC Psychiatry 24 , 232 (2024). https://doi.org/10.1186/s12888-024-05693-4
Download citation
Received : 19 October 2023
Accepted : 18 March 2024
Published : 27 March 2024
DOI : https://doi.org/10.1186/s12888-024-05693-4
Share this article
Anyone you share the following link with will be able to read this content:
Sorry, a shareable link is not currently available for this article.
Provided by the Springer Nature SharedIt content-sharing initiative
- Neurodevelopmental disorders
- Attention deficit hyperactivity disorder
- Autism spectrum disorder
- Tourette syndrome
- Mendelian randomization
- Fracture and osteoporosis
BMC Psychiatry
ISSN: 1471-244X
- Submission enquiries: [email protected]
- General enquiries: [email protected]

An official website of the United States government
The .gov means it’s official. Federal government websites often end in .gov or .mil. Before sharing sensitive information, make sure you’re on a federal government site.
The site is secure. The https:// ensures that you are connecting to the official website and that any information you provide is encrypted and transmitted securely.
- Publications
- Account settings
Preview improvements coming to the PMC website in October 2024. Learn More or Try it out now .
- Advanced Search
- Journal List
- v.43(2); 2018 Feb
Osteoporosis: A Review of Treatment Options
The authors review the pathophysiology, etiology, screening, and diagnosis of osteoporosis; selected professional guidelines and recommendations; nonpharmacological management; pharmacological options; and the cost-effectiveness of those options.
Approximately 10 million men and women in the U.S. have osteoporosis, 1 a metabolic bone disease characterized by low bone density and deterioration of bone architecture that increase the risk of fractures. 2 Osteoporosis-related fractures can increase pain, disability, nursing home placement, total health care costs, and mortality. 3 The diagnosis of osteoporosis is primarily determined by measuring bone mineral density (BMD) using noninvasive dual-energy x-ray absorptiometry. Osteoporosis medications include bisphosphonates, receptor activator of nuclear factor kappa-B ligand inhibitors, estrogen agonists/antagonists, parathyroid hormone analogues, and calcitonin. 3 – 6 Emerging therapies utilizing novel mechanisms include a cathepsin K inhibitor and a monoclonal antibody against sclerostin. 7 , 8 While professional organizations have compiled recommendations for the management of osteoporosis in various populations, a consensus has yet to develop as to which is the gold standard; therefore, economic evaluations have been increasingly important to help guide decision-makers. A review of cost-effectiveness literature on the efficacy of oral bisphosphonates has shown alendronate and risedronate to be most cost-effective in women with low BMD without previous fractures. 9 Guidelines are inconsistent as to the place in therapy of denosumab (Prolia, Amgen). In economic analyses evaluating treatment of postmenopausal women, denosumab outperformed risedronate and ibandronate; its efficacy was comparable to generic alendronate, but it cost more. 10 With regard to older men with osteoporosis, denosumab was also found to be cost-effective when compared with bisphosphonates and teriparatide (Forteo, Lilly). 11
INTRODUCTION
Osteoporosis is a bone disorder that increases a person’s risk of fracture due to low bone mineral density (BMD), impaired bone microarchitecture/mineralization, and/or decreased bone strength. This asymptomatic condition often remains undiagnosed until it manifests as a low-trauma fracture of the hip, spine, proximal humerus, pelvis, and/or wrist, which frequently leads to hospitalization. 4 , 12 The prevalence of osteoporosis is projected to rise in the United States from approximately 10 million people to more than 14 million people by 2020. 13 Although osteoporosis is typically associated with women, it is also diagnosed in men, who account for an estimated one in five of Americans who have osteoporosis or low BMD. 13 In addition to being the major cause of fractures in the older population, osteoporosis is also highly associated with people becoming bedridden, which can lead to serious complications. 14
In 2015, direct medical costs totaled $637.5 million for fatal fall injuries and $31.3 billion for nonfatal fall injuries. During the same year, hospitalizations cost an average of $30,550 per fall admission, totaling $17.8 billion. 15 By 2025, the cost of fractures in the United States is expected to exceed $25 billion each year to treat more than three million predicted fractures. 13 Management of osteoporosis and its associated consequences is necessary to improve quality of life and reduce economic burden on the health care system. It will also help to decrease medical visits, hospitalizations, and nursing home admission.
In recent years, major therapeutic advances in osteoporosis treatment have been made as scientists gain a greater understanding of bone morphology and the underlying mechanisms causing osteoporosis. This article will review the pathophysiology, etiology, screening, and diagnosis of osteoporosis; selected professional guidelines and recommendations; nonpharmacological management; pharmacological options; and the cost-effectiveness of those options.
PATHOPHYSIOLOGY
Bones provide structure for the body, protection for the organs, and storage for minerals, such as calcium and phosphorus, that are essential for bone development and stability. Individuals continue to build bone and will reach peak bone mass at about 30 years of age, after which they begin to lose bone mass steadily. Although peak bone mass is highly dependent upon genetics, many modifiable factors can influence bone mass, such as nutrition, exercise, and certain diseases and/or medications. 16
Throughout life, bones are remodeled, meaning that they are continuously resorbed by osteoclasts and replaced with new bone made by osteoblasts. This process allows for maintenance of mechanical strength and repair. An imbalance in remodeling activity in which resorption exceeds formation may result in the pathophysiological changes seen in osteoporosis. 17
Hormones and growth factors have a role in regulating bone function. Estrogen and testosterone have a significant effect on bone remodeling primarily by inhibiting bone breakdown. Cytokines that influence remodeling have also been identified, such as receptor activator of the nuclear factor kappa-B ligand (RANKL). RANKL is produced by osteoblasts that bind to RANK receptors on osteoclasts, leading to the activation and maturation of osteoclasts and culminating in bone resorption. 17 Recent advances in molecular bone biology have identified a potent protease named cathepsin K (CatK). CatK is secreted by activated osteoclasts during the bone resorption process, resulting in the degradation of bone matrix and breakdown of mineral components of bone tissue. 18 Parathyroid hormone (PTH) plays an important role in bone formation by indirectly increasing the proliferation of osteoblasts through regulation of calcium homeostasis. 18
Primary Osteoporosis
Primary osteoporosis is often associated with age and sex hormone deficiency. Age-related osteoporosis results from the continuous deterioration of the trabeculae in bone. In addition, the reduction of estrogen production in post menopausal women causes a significant increase in bone loss. In men, sex-hormone–binding globulin inactivates testosterone and estrogen as aging occurs, which may contribute to the decrease in BMD with time. 12 , 17 , 19 , 20
Secondary Osteoporosis
Secondary osteoporosis is caused by several comorbid diseases and/or medications. 19 Diseases implicated in osteoporosis often involve mechanisms related to the imbalance of calcium, vitamin D, and sex hormones. 16 , 17 For example, Cushing’s syndrome has been found to accelerate bone loss through excess glucocorticoid production. 21 In addition, many inflammatory diseases, such as rheumatoid arthritis, may require the patient to be on long-term glucocorticoid therapy and have been associated with secondary osteoporosis. 6 , 16 Notably, glucocorticoids are considered the most common medications linked to drug-induced osteoporosis. 6 , 16 BMD has been found to decline rapidly within three to six months of initiation of glucocorticoid therapy. 6 The American College of Rheumatology (ACR) has detailed recommendations to aid in guiding therapy selection for the prevention and treatment of glucocorticoid-induced osteoporosis (GIO). 6
Causes of secondary osteoporosis may differ between genders. For men, excessive alcohol use, glucocorticoid use, and hypogonadism are more commonly associated with osteoporosis. 22 For example, men receiving androgen-deprivation therapy (ADT) for prostate cancer are at increased risk of osteoporosis; Shahinian et al. found that 19.4% of those treated with ADT experienced a fracture compared with 12.6% of those who were not. 23 Tannenbaum et al. found that osteoporosis in 32.4% of women was attributed to secondary causes, most often hypercalciuria, malabsorption of calcium, hyperparathyroidism, vitamin D deficiency, hyperthyroidism, Cushing’s disease, and hypocalciuric hypercalcemia. Of note, disorders of calcium metabolism and hyperparathyroidism contributed to 78% of the secondary causes. 24
SCREENING AND DIAGNOSIS
Published osteoporosis screening guidelines vary greatly. In general, most organizations recommend that all adults older than 50 years of age with a history of fracture receive BMD screening. 3 , 4 , 12 , 19 The Preventive Services Task Force recommends BMD screening for all women 65 years of age and older and for younger women with equivalent or greater fracture risk when compared to healthy Caucasian women 65 years of age and older with no additional risk factors. 25 The Endocrine Society recommends screening all men 70 years of age and older and men 50 to 69 years of age who have additional risk factors for secondary osteoporosis. 5
The benefit of screening for early detection of osteoporosis was demonstrated in a trial by Barr et al. involving 4,800 women between 45 and 54 years of age who were randomized either to be screened or not screened for osteoporosis. After a nine-year follow-up, increased use of hormone replacement therapy and other osteoporosis treatments resulted in a 25.9% decrease in fracture risk compared with the control group. The authors concluded that the significant outcomes were due to screening for osteoporosis. 26
The gold standard for diagnosing osteoporosis utilizes BMD measurements, especially in the hip and lumbar spine with the dual-energy x-ray absorptiometry (DXA) device or the occurrence of nontraumatic hip or vertebral fractures. 3 , 4 , 27 Resulting T-scores are used to interpret BMD and to correlate results with fracture risk. For example, low BMD (or a highly negative T-score) is strongly correlated with a high fracture risk ( Table 1 ). There is a lack of consistent evidence from randomized clinical trials regarding the recommended optimal frequency of monitoring BMD during osteoporosis treatment. The National Osteoporosis Foundation (NOF) recommends monitoring BMD one to two years after initiation of treatment and every two years thereafter. Other recent studies, such as Gourlay et al. and Berry et al ., suggest testing at least every four years. 4 , 28 , 29 The North American Menopause Society (NAMS) states that repeated testing in untreated postmenopausal women is not recommended until two to five years have passed. NAMS authors also note that repeated testing in women receiving osteoporosis therapy may not be clinically useful until one to two years after treatment initiation. 18
T-Scores and WHO Diagnostic Criteria for Osteoporosis 4 , 14
WHO = World Health Organization.
Another diagnostic instrument, available in print or online, is a risk-assessment tool developed by the University of Sheffield in Great Britain called FRAX (Fracture Risk Assessment Tool). It takes into account risk factors such as age, race, alcohol use, gender, body mass index, smoking history, prior personal or parental history of fracture, use of glucocorticoids, secondary osteoporosis, rheumatoid arthritis, and femoral neck BMD measurements to predict the 10-year probability of hip fracture and other major osteoporotic fracture. 3 In addition, it assesses country-specific probabilities based on epidemiological data. This tool can be used in conjunction with other diagnostic tools, such as the DXA scan, to determine appropriate patients for treatment. 30
Nevertheless, FRAX has limitations, including that it is not validated for use with total hip or lumbar spine BMD, for ethnic minorities, for those receiving osteoporotic treatment, or for ages outside the specified range of 40 to 90 years. In addition, it does not include a history of falls as a risk factor due to the lack of a standardized metric or pharmaceutical evidence in reducing fracture risk based on fall history. Finally, it does not make recommendations on whom to treat. 31
SELECT GUIDELINES AND RECOMMENDATIONS
In a systematic review, Solomon et al. looked at 18 osteoporosis guidelines, among them those of the NOF, the ACR, and the American Association of Clinical Endocrinologists and American College of Endocrinology (AACE/ACE). Researchers noted several key differences among the guidelines they evaluated, such as inclusion of a review of economics; whether the literature used in developing the guidelines was formally graded; whether practice algorithms were included; sponsorship by a pharmaceutical manufacturer; methods and formatting; target patient populations; and recommendations on what to do with certain bone densitometry scores or bone formation/resorption markers. The researchers concluded that the guidelines present a relatively consistent set of recommendations and that the inconsistency among them is unlikely to contribute to the undertreatment of osteoporosis. Notably, the researchers did not offer an opinion as to which guideline is or should be preferred. 32
The following guidelines were selected for review due to their popularity in clinical practice for the treatment of osteoporosis in both men and women:
AACE/ACE 2016—Postmenopausal Osteoporosis
AACE/ACE provides evidence-based information for the management of postmenopausal osteoporosis (PMO). In those with no prior fragility fractures or with moderate fracture risk, alendronate, risedronate, zoledronic acid, or denosumab (Prolia, Amgen) are appropriate as first-line options, while ibandronate and raloxifene are considered alternatives. In those with prior fragility fractures or indicators of high fracture risk, denosumab, teriparatide (Forteo, Lilly), and zoledronic acid are recommended for first-line use, with alendronate and risedronate as alternatives. Indicators of high fracture risk include advanced age, frailty, glucocorticoids, very low T-scores, and increased fall risk. Teriparatide, denosumab, or zoledronic acid should be considered for those unable to use oral therapy. Raloxifene or ibandronate may be used as initial therapy for spine-specific efficacy. While sequential therapy of teriparatide followed by an antiresorptive medication is supported, combination therapy of osteoporosis medications for treatment or prevention of osteoporosis in postmenopausal women is not recommended due to limited availability of supportive data, increased cost, and potential increased side effects. 3
NAMS 2010—PMO
NAMS created an evidence-based position statement regarding management strategies for PMO. Strategies include identifying postmenopausal women at risk for fracture, implementing dietary and lifestyle changes to reduce modifiable risk factors, and initiating pharmacological therapy in those indicated. While bisphosphonates are recommended as first-line PMO treatment options, the authors note that raloxifene should be considered for younger postmenopausal women with osteoporosis or with low BMD because it prevents bone loss and reduces risk of vertebral fractures. In addition, teriparatide is suggested for those at high risk for fracture. Calcitonin is not recommended as a first-line option and can be considered for women who are more than five years beyond menopause. 18
Endocrine Society 2012—Men
The Endocrine Society formulated practice guidelines specifically for osteoporosis management in men. While the authors state that generic alendronate will often be preferred, they recommend zoledronic acid for men with a recent hip fracture, nonoral therapy for those with gastrointestinal problems, and teriparatide for men at high risk for fracture because it increases spine BMD more than alendronate. In addition, researchers also suggested the consideration of risedronate as an alternative agent for men at risk for hip fractures. 5
ACR 2017—Glucocorticoid-Induced Osteoporosis
In GIO, individuals are stratified based on their age, fracture risk, and the dose and duration of glucocorticoid therapy. For all patients starting long-term glucocorticoid treatment, initial clinical fracture risk must be assessed and re-evaluated every 12 months. In general, the ACR recommends that for postmenopausal women and for men 40 years of age and older, as well as adults 30 years of age and older using high-dose glucocorticoids (prednisone equivalent dose of 30 mg or more per day or annual cumulative dose greater than 5 g), treatment with a bisphosphonate is preferred over teriparatide, denosumab, or raloxifene. 6
NOF 2014—PMO and Men at Least 50 years of Age
The NOF has developed a Clinician’s Guide to Prevention and Treatment of Osteoporosis. General considerations are included for women and men of varying age groups and generally parallel those of other prominent organizations. 4 Although this guidance provides general recommendations on diagnosis and screening, it does not provide recommendations for initial medication therapy or express a preference for one therapeutic class over another.
American College of Physicians 2017—Women And Men With Low BMD and Osteoporosis
The American College of Physicians (ACP) recently published updated treatment guideline recommendations for men and women with low BMD and osteoporosis. For women, pharmacological treatment with alendronate, risedronate, zoledronic acid, or denosumab for five years is appropriate. Authors specifically recommend against treatment with menopausal estrogen therapy, treatment with menopausal estrogen plus progestogen therapy, or raloxifene, and against BMD monitoring during the five-year treatment period. For women with osteoporosis who are at least 65 years of age and have a high risk for fracture, treatment decisions should be individualized; risk versus benefit, patient preferences, fracture-risk profile, and costs should be assessed to determine if osteoporotic treatment is warranted.
For men with osteoporosis, pharmacological treatment with bisphosphonates is recommended; there is no preference for a specific agent. Of note, authors made this recommendation based on extrapolation of data from studies done with women because data for men are sparse. 33
NONPHARMACOLOGICAL MANAGEMENT
Nonpharmacological management of osteoporosis includes adequate calcium and vitamin D intake, weight-bearing exercise, smoking cessation, limitation of alcohol/caffeine consumption, and fall-prevention techniques. 2 – 6 , 9 , 18 , 34
The Institute of Medicine (IOM) recommends that dietary calcium intake should be limited to 1,000 mg daily for men 50 to 70 years of age and to 1,200 mg daily for women 51 years of age and older and for men 71 years of age and older. 35 Published literature on calcium and the risk of developing kidney stones is controversial, so it is important to differentiate the effects of dietary calcium and supplemental calcium from vitamins. 36 High intake of calcium from supplements may increase the risk of kidney stones; however, high intake of dietary calcium may protect against kidney stones. 37 Therefore, it is recommended that dietary calcium intake be increased first before initiating calcium supplements to meet calcium requirements. 4
The relationship between calcium intake and cardio vascular risk has also been debated. A systematic review and meta-analysis funded by the NOF and the American Society of Preventive Cardiology concluded that dietary and supplemental calcium intake that does not exceed the upper limit recommended by the IOM poses neither cardiovascular risk or harm (myocardial infarction, stroke, or death) nor benefit for generally healthy adults. 38 , 39
Vitamin D is a key component in calcium absorption and bone health. The IOM recommends 600 IU per day for men and women 51 to 70 years of age and 800 IU per day for men and women older than 70 years. 35 Although some evidence supports using vitamin D supplementation to reduce fracture risk, recent studies have shown that higher monthly doses of vitamin D are associated with an increased risk of falls. This may warrant recommending lower daily doses of vitamin D. 40 , 41
PHARMACOLOGICAL TREATMENT
The goal of pharmacological therapy is to reduce the risk of fractures. 2 – 4 Medications to treat osteoporosis are categorized as either antiresorptive (i.e., bisphosphonates, estrogen agonist/ antagonists [EAAs], estrogens, calcitonin, and denosumab) or anabolic (i.e., teriparatide). Antiresorptive medications primarily decrease the rate of bone resorption while anabolic medications increase bone formation more than bone resorption. While several medications have overlapping indications, it is important to note that not all osteoporosis medications are approved by the Food and Drug Administration (FDA) to treat PMO, osteoporosis in men, and/or GIO ( Table 2 ). Per AACE/ACE guidelines, first-line treatment for most PMO patients at high risk of fracture includes alendronate, risedronate, zoledronic acid, and denosumab. For those who cannot use oral therapy and are at high risk of fracture, use of teriparatide, denosumab, or zoledronic acid is recommended. 3 This recommendation is also reflected in the ACP guidelines, and authors notably suggest treatment duration of five years for PMO, as well as first-line treatment with bisphosphonates for men with osteoporosis. 33
Food and Drug Administration-Approved Indications for Osteoporosis Treatments 42 – 46 , 49 – 51 , 71 , 75 , 78 , 84 – 86 , 88 , 89 , 93 , 97
GIO = glucocorticoid-induced osteoporosis; PMO = postmenopausal osteoporosis.
Recommendations for treatment options are based on different characteristics, such as gender, degree of fracture risk, and additional risk factors, such as comorbid diseases or medications. 3 – 6 The AACE/ACE recommends that pharmacological treatment should be initiated for: 1) patients with osteopenia or low bone mass and a history of fragility fracture at the hip or spine; 2) patients with a T-score of −2.5 or less in the lumbar spine, femoral neck, total hip, or 33% radius despite the absence of a fracture; or 3) patients with a T-score between −1.0 and −2.5 if the FRAX 10-year probability for a major osteoporotic fracture is greater than 20% or for a hip fracture is greater than 3%. 3 The NOF and Endocrine Society suggest similar guidelines for the diagnosis and initiation of treatment. 4 , 5
Antiresorptive Agents
Bisphosphonates.
AACE/ACE, ACR, NAMS, and the Endocrine Society recommend bisphosphonates, excluding ibandronate, as a first-line option for the prevention and/or treatment of osteoporosis in postmenopausal women, men, and/or GIO patients ( Table 2 ). 3 , 5 , 6 , 18 Bisphosphonates bind with high affinity to the mineral matrix of the bone and inhibit osteoclast resorption of the bone, leading to a decrease in bone turnover and a net gain in bone mass. 42 – 49 Alendronate, risedronate, and zoledronic acid (intravenous [IV]) have demonstrated an increase in BMD and a decrease in risk of fractures due to osteoporosis in men, postmenopausal women, and GIO patients. 50 – 59 Ibandronate is not a first-line recommendation even though high-quality evidence indicates that it reduces vertebral fractures in both men and women; there is insufficient evidence to determine its effect on hip fractures. In addition, there is strong evidence that it has no effect on nonvertebral fracture risk. 33 , 60
Bisphosphonates are available in multiple formulations. Alendronate, risedronate, and ibandronate are available as oral tablets. 42 – 45 Alendronate is also available as effervescent tablets (Binosto, Mission Pharmacal Co.) and a combination formulation with vitamin D (Fosamax Plus D, Merck). 46 , 47 Risedronate is available as immediate release or delayed release; of note, two studies concluded that the delayed-release formulation is noninferior to immediate release. 48 , 49 Zoledronic acid and ibandronate are available as IV injections. 50 – 51 Doses for each agent depend upon whether prophylactic or treatment doses are being recommended. Most formulations also utilize extended-interval dosing, such as once weekly or monthly, due to the long half-lives of these agents. Bisphosphonates are excreted by the kidneys; thus, toxicities may occur from accumulation in patients with renal impairment. Therefore, bisphosphonates should be avoided in patients whose creatinine clearances fall below established recommendations ( Table 3 ). 42 , 43 , 45 , 46 , 50 , 51
Dose Recommendations for Bisphosphonates 42 , 43 , 45 , 56 , 48 , 49
CrCl = creatinine clearance; IR = immediate release; IV = intravenous; PO = orally.
Oral bisphosphonates should be administered with a full glass of water in the morning on an empty stomach 30 minutes prior to a meal or other medications (60 minutes for ibandronate). Patients should remain upright for at least 30 minutes post-dose to prevent esophageal irritation. 42 – 47 These recommendations aim to increase agents’ bioavailability and prevent adverse drug reactions. For example, the most notable adverse drug reaction associated with oral bisphosphonates is upper gastrointestinal discomfort, which may include heartburn, indigestion, esophageal erosion, and esophageal ulcer. Acute-phase injection reactions (e.g., fever, muscle aches) have been associated with use of IV formulations and may require pretreatment with oral acetaminophen. 3
All bisphosphonates are reported to be associated with a rare complication called osteonecrosis of the jaw (ONJ), defined as the presence of exposed and necrotic bone in the maxillofacial region that does not heal within eight weeks. 63 ONJ has been observed in patients receiving prolonged bisphosphonate therapy who undergo invasive dental procedures, such as tooth extractions. Among the bisphosphonates, a higher incidence of ONJ has been seen with zoledronic acid. 42 – 46 , 49 – 51 Another rare complication reportedly associated with bisphosphonate use is increased risk of low-trauma atypical femur fractures (AFFs). In 2010, the FDA released a safety communication stating that it is unclear whether bisphosphonates are the cause of atypical subtrochanteric femur fractures and/or diaphyseal femur fractures, but that they may be related to long-term use; therefore, while the optimal treatment duration of bisphosphonate therapy is unknown, it is important to consider either discontinuation or a drug holiday when the risks of use outweigh the benefits. 64 Because bisphosphonates may accumulate in bone and continue to be released for months or years after treatment cessation, drug holidays or treatment interruptions can be considered in appropriate patients. 67 For patients at moderate to lower fracture risk, a drug holiday can be considered after three to five years of oral bisphosphonate use or after three annual doses of IV zoledronic acid. 3 , 68 For patients at higher fracture risk, drug holidays can be considered after six to 10 years of oral bisphosphonate use or after six annual doses of IV zoledronic acid. Patients who are at higher risk could also consider using teriparatide or raloxifene during drug holidays. 3 The optimal duration of a drug holiday has not yet been established, but longer duration of use of bisphosphonates with a higher binding affinity to bone (zoledronic acid is greater than alendronate, which is greater than risedronate) has been suggested. 3 , 65 Providers may consider restarting therapy if the patient experiences fracture, shows significant BMD loss, or has a rise in bone turnover markers (BTMs) to pretreatment levels. 3 Notably, in a study by Bauer et al., the authors found that follow-up DXA measurements one year after alendronate cessation, and two biomarkers of bone turnover assessed one to two years after treatment cessation, were not associated with fracture risk. The authors recommended against assessing these measurements during an alendronate holiday. 69 Ultimately, the decision to restart osteoporotic treatment following a drug holiday should be done on an individualized basis with a proper assessment of risks and benefits by a clinician.
The Fracture Intervention Trial (FIT) demonstrated significant reductions in the incidence of vertebral fractures in postmenopausal women treated with alendronate 10 mg per day for three to four years with existing fractures or a femoral T-score of less than −2.5. 70 The Fracture Intervention Trial Long-Term Extension was a continuation of FIT comparing the duration of treatment in postmenopausal women receiving alendronate for five years versus 10 years. Women who discontinued alendronate after five years did not show a significant difference in nonvertebral fractures (18.9% for placebo versus 19% for alendronate; 95% confidence interval [CI], 0.76–1.32). There was a significantly lower risk in clinically recognized vertebral fractures for those who continued alendronate (5.3% for placebo versus 2.4% for alendronate; 95% CI, 0.24–0.85), but no significant difference in morphometric vertebral fractures (11.3% for placebo versus 9.8% for alendronate; 95% CI, 0.60–1.22). Thus, the authors concluded that for most women, five years of treatment with alendronate was sufficient to maintain bone mass and reduce bone remodeling; however, women who have very low BMD and/or a very high risk of developing vertebral fractures may benefit from continuing alendronate beyond five years. 71
The AACE/ACE recommends denosumab as first-line therapy for patients at high risk of fracture and for patients who are unable to use oral therapy. 3 Denosumab was the first biologic agent available for treatment of osteoporosis. It is a fully human monoclonal antibody that inhibits RANKL to decrease bone resorption. RANKL is a transmembrane protein required for the formation, function, and survival of osteoclasts. 72 Denosumab is FDA approved for the treatment of PMO with high risk for fracture, as well as for women with breast cancer receiving adjuvant aromatase inhibitor therapy. It has also been approved for the treatment of bone loss in men with osteoporosis and with prostate cancer receiving ADT. 72 – 74 The FREEDOM trial enrolled 7,868 postmenopausal women with osteoporosis and demonstrated that 60 mg denosumab every five months for 36 months significantly reduced the risk of hip, nonvertebral, and vertebral fractures compared with placebo. In 36 months, reduction in the relative risk of new radiographic vertebral fractures, clinically diagnosed vertebral fractures, and multiple new vertebral fractures was 68%, 69%, and 61%, respectively, with denosumab use ( P < 0.001 for both comparisons). In addition, the relative risk reduction of non vertebral fractures and hip fractures was 20% ( P = 0.01) and 40% ( P = 0.04), respectively, when compared with placebo. Denosumab use also increased BMD at the lumbar spine by 9.2% (95% CI, 8.2–10.1) and at the total hip by 6% (95% CI, 5.2–6.7). 75
Denosumab is available as an injectable formulation in either a prefilled syringe or a single-use vial. The treatment dose for osteoporosis is 60 mg subcutaneously (SC) every six months administered by a health care professional. Denosumab is well tolerated, but reported adverse effects include hypersensitivity, serious infections, dermatological reactions, musculo skeletal pain, and hypercholesterolemia. Denosumab can cause hypocalcemia, so calcium levels should be corrected prior to treatment initiation. Rare cases of ONJ and AFF associated with prolonged use of denosumab have also been reported. Dosage adjustments are not recommended for denosumab in patients with renal or hepatic impairment, but a significant risk of hypocalcemia occurs in patients with severe renal impairment (creatinine clearance less than 30 mL/min). Denosumab is safe in patients with chronic kidney disease (CKD) stages 1 to 3 but is not recommended for use in patients on dialysis or with stage 5 CKD. 3 , 72 Notably, per the AACE/ACE 2016 guidelines, a drug holiday is not recommended with denosumab because treatment cessation was associated with a decrease in BMD after two years and an increase in BTMs after one year. 3
Hormonal Therapies
Estrogen agonist/antagonists.
This class of drugs is also known as selective estrogen receptor modulators (SERMs).
Raloxifene, which is characterized as an EAA, exhibits dual agonistic and antagonistic properties in estrogenic pathways. Raloxifene acts as an estrogenic agonist on the bone by decreasing bone resorption and bone turnover, thus increasing BMD. It also has estrogen antagonistic activity on breast and uterine tissue. The AACE/ACE recommends raloxifene as an appropriate first-line therapy for patients requiring reduced risk of spine fracture only. Due to its selective antagonistic effects on breast tissue, raloxifene may be considered in women with an increased risk of vertebral fractures who may be at risk for developing breast cancer. 76 Raloxifene can also be used as a weaker antiresorptive therapy for higher-risk patients during a bisphosphonate holiday. 3 The MORE study was a multicenter, randomized, blinded, placebo-controlled trial of 7,705 women diagnosed with osteoporosis who had been post-menopausal for at least two years. The results demonstrated a four-year cumulative relative risk of 0.7 (95% CI, 0.5–0.8) for new vertebral fractures and relative risk of 0.9 (95% CI, 0.8–1.1) that was not significant for nonvertebral fractures. 77 A substudy of the MORE trial by Ettinger et al. reported an increase in BMD of 2.1% and 2.6% at the femoral neck and spine, respectively, in women who received raloxifene 60 mg per day compared with women who received placebo. The relative risk of 0.7 (95% CI, 0.5–0.8) was significant for vertebral fractures and the relative risk of 0.9 (95% CI, 0.8–1.1) was not significant for nonvertebral fractures in women receiving raloxifene 60 mg per day. 78
Raloxifene is dosed at 60 mg per day without regard to food. Adverse events reported in clinical trials included vaginal bleeding, hot flashes, worsening of pre-existing hypertriglyceridemia, venous thromboembolism (VTE, including deep vein thrombosis and pulmonary embolism), death due to stroke (specifically in women with documented coronary heart disease or at increased risk for major coronary events), and cardiovascular disease. Raloxifene should be avoided in women who have a history of or active VTE, who are premenopausal, who are pregnant or may become pregnant, or who are breastfeeding. 76
Conjugated Estrogens/Bazedoxifen
A combination of conjugated estrogens with bazedoxifene (Duavee, Pfizer) received FDA approval in 2013 for use in postmenopausal women with an intact uterus for the prevention of osteoporosis and for the treatment of moderate-to-severe vasomotor symptoms. Duavee is sometimes referred to as a tissue-selective estrogen complex. 4 Bazedoxifene acts as an EAA to reduce the risk of endometrial hyperplasia associated with the estrogen component. 79 Based on a study by Silverman et al., bazedoxifene 20 mg monotherapy can reduce the risk of vertebral fracture by 42% (hazard ratio [HR], 0.58; 95% CI, 0.38–0.89) and combined data for bazedoxifene 20 mg and 40 mg showed that they reduced nonvertebral fractures in women at higher risk by 40% (HR, 0.60; 95% CI, 0.37–0.95) compared with placebo. 80 FDA approval was based on three clinical trials demonstrating that Duavee reduced hot flashes and increased BMD at the hip and spine in postmenopausal women compared with placebo. Due to a lack of fracture data, the actual efficacy of Duavee for PMO remains unclear. There were, however, significant reductions in serum BTMs from baseline with all conjugated estrogens/bazedoxifene doses compared to placebo ( P < 0.001). 79 – 84
Duavee tablets contain 0.45 mg conjugated estrogens and 20 mg bazedoxifene and are dosed once daily. Its clinical role in therapy is for the prevention of osteoporosis with the additional indication of treating vasomotor symptoms, but careful consideration should be exercised because it has the same boxed warnings, precautions, and contraindications as other estrogen-containing medications. 79
Estrogen-Progestin Therapy
In terms of osteoporotic management, estrogen therapy is FDA approved solely for the prevention of osteoporosis in high-risk postmenopausal women and should be used only after all nonestrogenic osteoporotic treatments have been considered inappropriate. 85 – 87
The Women’s Health Initiative (WHI) was a randomized controlled trial of 16,608 postmenopausal patients that demonstrated statistically significant reduction in fractures with estrogen-progestin combination therapy; however, the WHI study data also reported an increase in the risk of cardiovascular events, stroke, VTE, and invasive breast cancer associated with the estrogen-progestin groups. 88 Due to the overall health risks exceeding benefits, hormonal replacement therapy is no longer recommended as first line for the treatment and prevention of osteoporosis in postmenopausal and premenopausal women. 3
Testosterone Therapy
Despite limited studies involving the use of such combinations, the Endocrine Society recommends combination use of antifracture treatment with testosterone therapy for men at high risk of fracture. Testosterone monotherapy is recommended either for those in whom antiosteoporotic therapy is contraindicated and whose testosterone levels are less than 200 ng/dL, or for those at borderline high risk for fracture who have serum testosterone levels less than 200 ng/dL and have signs or symptoms of androgen deficiency or hypogonadism. 5
Calcitonin is a synthetic polypeptide hormone with properties similar to natural calcitonin found in mammals, birds, and fish. The effects of calcitonin on normal human bone physiology are unclear; however, calcitonin receptors have been discovered on osteoclasts and osteoblasts. 89 , 90 Calcitonin is FDA approved for the treatment of osteoporosis in women who have been postmenopausal for more than five years when alternative treatments are not feasible. Results for a five-year, double-blind, randomized, placebo-controlled study of 1,255 postmenopausal women with established osteoporosis indicated that 200 IU of calcitonin daily reduced the risk of new vertebral fractures by 33%. 89 Unlike bisphosphonates and denosumab, calcitonin lacks data showing a reduction in nonvertebral fractures, thus it is not considered first-line treatment for osteoporosis. 92
Calcitonin-salmon nasal spray is available only as a generic and is administered as one spray in one nostril daily, alternating nostrils. 92 Miacalcin nasal spray and Fortical nasal spray (calcitonin-salmon, rDNA origin) are no longer on the market. 89 , 90 Miacalcin SC injection is available but rarely used. The most common adverse reactions seen with use include rhinitis, nasal irritation, back pain, arthralgia, nosebleed, and headache. Patients older than 65 years of age may have a higher risk for nasal adverse reactions. Skin testing may be considered prior to treatment for those with suspected sensitivity to calcitonin because serious allergic reactions have been reported. In 2013, an FDA long-term post-marketing review suggested a very modest increase in cancer rates among calcitonin-treated patients and recommended that health care professionals assess the use of calcitonin for osteoporosis therapy versus other available treatments. 93
Parathyroid Hormone Analogues
Teriparatide.
Teriparatide, a recombinant human PTH (1–34) analogue, is the first anabolic treatment approved for osteoporosis. It mimics the physiological actions of PTH in stimulating new bone formation on the surface of bone by stimulating osteoblastic activity when given intermittently at small doses. 95 The AACE/ACE suggests the use of teriparatide for initial PMO treatment in those with prior fragility fractures or with high fracture risk and for those who are unable to take oral therapy. It is also listed as an option for higher-risk patients on bisphosphonate holiday. 3
Neer et al. studied the effects of teriparatide 20 mcg, teriparatide 40 mcg, and placebo in 1,326 postmenopausal women with osteoporosis for an average of 21 months. The study reported a decrease in new vertebral and nonvertebral fractures with increases in vertebral, femoral, and total-body BMD in women using teriparatide. 95 Saag et al. compared the efficacy of teriparatide with alendronate in 428 men and women 22 to 89 years of age with GIO in an 18-month, randomized, double-blind trial. Researchers reported an increase in BMD in the spine and hip with significantly fewer new vertebral fractures in patients using teriparatide versus alendronate in those at high risk for fracture; there was no significant difference in the two groups in the incidence of nonvertebral fractures. 96
The FDA-recommended dose of teriparatide is 20 mcg SC once daily in the thigh or abdomen. The duration of therapy is limited to two years due to the development of osteosarcoma in rats at high doses. 94 Of note, a seven-year interim analysis (2004–2011) from a 15-year ongoing post-marketing surveillance study analyzing the correlation between osteosarcoma and the use of teriparatide in humans did not demonstrate a causal association. 97 The AACE/ACE recommends treatment with an antiresorptive agent immediately following teriparatide therapy to avoid bone density decline. 3 Teriparatide should be avoided in patients with Paget’s disease of bone, unexplained alkaline phosphatase elevations, prior skeletal radiotherapy, primary or metastatic bone malignancy, or hypercalcemic disorders, such as primary hyperparathyroidism. 94
Abaloparatide
Abaloparatide (Tymlos, Radius Health), the second recombinant human PTH (1–34) analogue to reach the market, received FDA approval in April 2017. 98 It is indicated for the treatment of PMO in women at high risk for fracture, defined as a history of osteoporotic fracture or multiple risk factors for fracture, and in patients who have failed or are intolerant to other available osteoporosis therapy. In a phase 3 clinical trial, abaloparatide reduced the incidence of new vertebral fracture by 86% over an 18-month period. The drug also reduced the risk for nonvertebral fracture by 43%. 99
Abaloparatide is available as an injection. The recommended dose is 80 mcg SC once daily into the periumbilical region of the abdomen. Abaloparatide carries the same boxed warning as teriparatide: The duration of therapy is limited to two years due to the development of osteosarcoma in rats. 89 However, one possible advantage of abaloparatide over teriparatide is cost. At the current list price, a 30-day supply of the abaloparatide injector pen costs approximately half as much as the teriparatide pen. 100 Of note, abaloparatide also carries a risk of orthostatic hypotension, hypercalcemia, and urolithiasis. Use is to be avoided in those with pre-existing hypercalcemia and those with an underlying hypercalcemic disorder, such as primary hyperparathyroidism. The most common adverse reactions seen with use in clinical trials were dizziness, nausea, headache, palpitations, fatigue, upper abdominal pain, and vertigo. 98
Emerging Therapies and Investigational Drugs
Romosozumab.
Romosozumab (Evenity, Amgen/UCB) is a humanized monoclonal antibody that inhibits sclerostin. In the skeletal tissue, sclerostin is a protein secreted by osteoclasts to reduce bone formation by interfering with the proliferation and function of osteoblasts. The international, 24-month FRAME trial compared romosozumab with placebo in 7,180 postmenopausal women with a T-score of −2.5 to −3.5 at the total hip or femoral neck. Patients received SC romosozumab 210 mg or placebo once monthly for 12 months during the double-blind phase of the trial. Then, all patients received open-label denosumab, administered SC at 60 mg per dose every six months for an additional 12 months. The results showed that patients who received romosozumab had a 73% lower risk of new vertebral fracture at 12 months compared with placebo (incidence, 0.5% versus 1.8%; relative risk, 0.27; 95% CI, 0.16–0.47; P < 0.001); however, there was no significant difference in the risk of nonvertebral or clinical fracture at 24 months. Romosozumab increased BMD at the lumbar spine, total hip, and femoral neck by 13.3%, 6.9%, and 5.9% respectively ( P < 0.001 for all comparisons). 101
As of July 2017, the FDA had rejected approval of romosozumab for osteoporosis treatment due to a higher rate of serious adverse cardiovascular events compared with alendronate. Amgen and UCB are pooling late-phase data and refiling their application in an effort to show the drug has a positive risk–benefit profile. 102
Other antisclerostin monoclonal antibodies being developed and tested include blosozumab and BPS804. 103
Odanacatib is a selective inhibitor of CatK, a protease that is released by osteoclasts to promote the degradation of collagen in bones. Inhibiting CatK is theorized to decrease bone resorption without decreasing bone formation. In 2016, Merck discontinued development of odanacatib due to an increased risk of stroke. 104
Lasofoxifene
Lasofoxifene (Sermonix) is a third-generation SERM. The PEARL trial studied the effects of lasofoxifene in an inter national, randomized, placebo-controlled trial of 8,556 women between 59 and 80 years of age who had a BMD T-score of 2.5 or less at the femoral neck or spine. Participants received either 0.25 mg or 0.5 mg lasofoxifene daily versus placebo for five years. The group that received the clinically approved dose of lasofoxifene 0.5 mg per day demonstrated a relative risk reduction of 42% and 24% in vertebral fractures and nonvertebral fractures, respectively. Researchers also found that therapy was associated with reductions in breast cancer, coronary heart disease, and stroke. 105 Lasofoxifene is approved for osteoporosis treatment in Europe, but approval is pending in the U.S. 106
COST-EFFECTIVENESS ANALYSIS
Due to the substantial growth of the aging population and the likely increase in osteoporosis incidence, several studies have sought to clarify the treatment thresholds at which osteoporosis treatment becomes cost-effective. While other cost-effectiveness analyses have been conducted, the following were included due to their focus on cost-effectiveness from a U.S. perspective. Studies performed in other countries with universal or socialized health care may not reflect U.S. costs.
An NOF-supported economic analysis by Tosteson et al. created a Markov-cohort model to determine the absolute 10-year fracture risk at which osteoporosis treatment became cost-effective. Willingness to pay was defined at $60,000 per quality-adjusted life year (QALY) gained. This analysis produced an absolute 10-year hip fracture probability of 3% for women and 3.5% for men as the treatment intervention threshold—that is, treatment becomes cost-effective only once a patient’s 10-year fracture risk meets or exceeds this threshold. The authors noted that for groups 65 years of age and older, this fracture risk threshold tended to increase. Although the authors presented the results in the form of a 10-year hip-fracture probability, they also accounted for the impact of fractures at other sites. This analysis also examined how alterations in annual treatment cost and willingness-to-pay thresholds changed the intervention thresholds. The authors found that annual treatment cost (ranging from $300 to $900) had more impact on the variation in the intervention threshold than the willingness-to-pay threshold (ranging from $50,000 to $75,000). A disadvantage of this analysis was that the annual cost of treatment for some first-line agents exceeded the $600 used in the author’s base-case analysis, as seen in Table 4 ; in addition, this analysis assumed 100% treatment compliance over a five-year period, which is not realistic. 107
AWPs of Selected Osteoporosis Medications 110
AWP = average wholesale price; DR = delayed release; IN = intranasal; IV = intravenous; PO = oral; RANKL = receptor activator of nuclear factor kappa-B ligand; SC = subcutaneous.
In incidence-based Markov modeling by Tosteson et al. that evaluated the cost-effectiveness for PMO in the United States, risedronate provided the most benefit in terms of QALYs gained and hip fractures averted at the lowest cost for all patient risk groups. In women 65 years of age with a previous fracture, the incremental cost-effectiveness ratio (ICER) was $22,068 per QALY gained and $45,865 per hip fracture averted. This was considered cost-effective because researchers set a decision threshold of $50,000 per QALY compared to no therapy. In comparison, the ICER value was $362,945 per QALY gained for alendronate. While pharmacological treatment was expected to be more expensive than no treatment, risedronate produced cost-savings compared to no therapy for women 75 years of age with a previous fracture. Researchers also evaluated ibandronate and teriparatide, concluding that their use was associated with a higher cost and a poorer outcome in all patient risk groups compared to no treatment. The cost-effectiveness results changed with alterations in the assumption of treatment efficacy and time horizon. 9
A Markov model by Parthan et al. comparing oral bisphosphonates with denosumab in the U.S. PMO population found that, overall, denosumab dominated branded risedronate (Actonel, Warner Chilcott) and branded ibandronate (Boniva, Roche). Denosumab had a cost-effective ICER of $85,100 per QALY compared to alendronate, using a cost-effectiveness threshold of $100,000 per QALY. In several analyses of high-risk subgroups among women 75 years of age and older, denosumab outperformed all oral bisphosphonates. The authors also examined a high-risk subgroup that had two or more of the following risks: older than 70 years of age, BMD T-score of −3.0 or less, and prevalent vertebral fracture. Again, denosumab overshadowed Actonel and Boniva with a cost-effective ICER of $7,900 per QALY compared to alendronate. The disadvantage of this study was its use of branded risedronate and ibandronate, both of which are now available as lower-cost generics. Thus, while denosumab dominated branded risedronate and ibandronate due to its lower cost and higher QALYs, this conclusion may now be inaccurate due to cost changes. 10
In a microsimulation model, Liu et al. compared teriparatide with alendronate in women with severe osteoporosis (defined as low bone mass and pre-existing fractures). They analyzed three treatment strategies compared with usual care (defined as calcium or vitamin D supplementation). The three approaches were five years of alendronate, two years of teriparatide, and two years of teriparatide followed by five years of alendronate (sequential therapy). The base case analysis produced an ICER of $11,600 per QALY for alendronate alone compared with usual care and $156,500 per QALY for sequential therapy compared with alendronate. Both strategies outperformed teriparatide monotherapy because it cost more and increased QALYs less than alendronate. In further sensitivity analyses, the cost-effectiveness of sequential therapy generally improved with increasing age and decreasing femoral neck BMD, except among women 70 to 80 years of age. Cost-effectiveness for sequential therapy was projected to decrease to less than $50,000 per QALY in female PMO patients with exceptionally low femoral neck T-scores (−4.0 or less) and prior vertebral fractures. This analysis used branded alendronate; generic alendronate would likely be more cost-effective compared to sequential therapy or teriparatide alone. Researchers modeled the analysis with treatment-naïve women, which may not be realistic for this population considering their diagnoses of severe osteoporosis and pre-existing fractures. 108
With regard to older U.S. men with osteoporosis, a study by Silverman et al. concluded that denosumab is the most cost-effective treatment compared with bisphosphonates (alendronate, risedronate, ibandronate, zoledronate) and teriparatide. Researchers adapted a previously published lifetime cohort Markov model to study men 75 years of age and older. Although alendronate was associated with the lowest lifetime costs, men using denosumab had 0.05 additional QALYs, producing an ICER of $16,900 compared with alendronate and dominating the other comparators. The ICER was sensitive to changes in the relative risk of hip fracture with denosumab/alendronate, the drug cost of denosumab, and the unit cost of one day in a nursing home. Overall, those on denosumab had the lowest 10-year risk of hip fractures. This article had several limitations: authors used data from PMO trials to build their Markov model, the Markov model assumed that once patients experienced a fracture they would not have another milder fracture, and the model’s target population was derived from the ADAMO trial, which is not representative of all male osteoporotic patients. 11
The Institute for Clinical and Economic Review released an assessment of the cost-effectiveness of abaloparatide and teriparatide in June 2017. In its simulation model, two years of therapy with either abaloparatide or teriparatide was followed by six years of treatment with zoledronic acid; this was compared to treatment with zoledronic acid alone. The target population was 70-year-old women at high risk for osteoporotic fractures. QALYs gained versus zoledronic acid were 0.066 for abaloparatide and 0.046 for teriparatide over the lifetime horizon. Incremental costs versus zoledronic acid ranged from $22,061 for abaloparatide to $43,440 for teriparatide, despite estimated price discounts of 27% and 38%, respectively, for the anabolic therapies. The base case ICERs for each anabolic drug compared to zoledronic acid greatly exceeded the commonly cited cost-effectiveness threshold of $150,000 per QALY. Notable limitations include possible underestimation of the number of less-severe fractures compared with prior fractures; lack of consideration of adverse events; an assumption of 100% adherence; and authors’ assumptions about drug prices. 100
Osteoporosis is a worldwide concern, causing more than 8.9 million fractures per year. 109 The expected increase in medical visits, hospitalizations, and nursing home placements related to osteoporotic fractures will contribute to a substantial economic burden on health care systems. Thus, screening is important based on age, gender, and other risk factors. Bisphosphonates remain the first-line and most cost-effective treatment option for osteoporosis, but there is increasing concern about their long-term safety. Medications with novel mechanisms to treat osteoporosis can be expected in the near future. 3 – 6 Although appropriate BMD screening and treatment with medication is important, osteoporosis is preventable with proper management of diet, lifestyle, and fall prevention interventions.
ACKNOWLEDGEMENT
The authors would like to acknowledge the contributions of Samuel S. Murray, MD.
Disclosures: The authors report no commercial or financial interests in regard to this article.
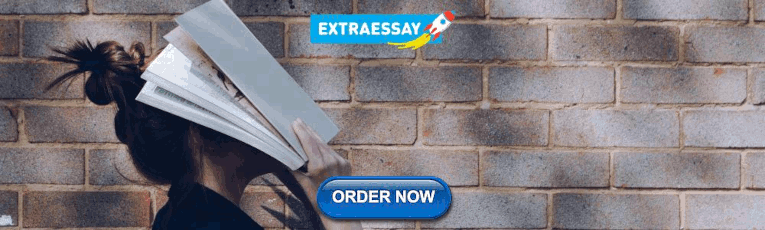
IMAGES
VIDEO
COMMENTS
Osteoporosis is a metabolic bone disorder characterized by progressive decline of bone mass and bone quality, leading to bone fragility and an increased risk of fracture. ... New research shows ...
In the United States, approximately 20% of women over 50 years of age and 30% of women 65 years of age or older meet DXA criteria for osteoporosis. 1,2 In the United States, osteoporosis is more ...
What is osteoporosis? Learn about osteoporosis symptoms, prevention and treatment. Find out the causes of osteoporosis and the effect of diet, exercise and drugs. Find the latest medical research ...
Español. The U.S. Food and Drug Administration today approved Evenity (romosozumab-aqqg) to treat osteoporosis in postmenopausal women at high risk of breaking a bone (fracture). These are women ...
The study opens the door to new treatments and preventive measures for bone regeneration and osteoporosis research. ... New osteoporosis therapy's dual effects on bone tissue. Jun 25, 2019.
A new discovery about osteoporosis suggests a potential treatment target for that brittle-bone disease and for bone loss from rheumatoid arthritis. Your source for the latest research news
Other research interests include the mechanisms and treatment of the loss of cortical bone as it is more closely related to osteoporotic fractures. Aging is also an important factor for OP. ... New Frontiers in Osteoporosis Therapy. Annu. Rev. Med. 71, 277-288. 10.1146/annurev-med-052218-020620 [Google Scholar] Claudel M., Jouzeau J. Y ...
Advances in imaging techniques of osteoporosis. For epidemiological purposes, osteoporosis was defined by World Health Organization (WHO) as 'BMD lower than -2.5 SD below the peak bone mass of young healthy adults'. 18 While the WHO definition is widely utilized in daily clinical practice to make a diagnosis of osteoporosis, it should be recognized that it is plagued by several disadvantages.
A new aid to osteoporosis and fracture risk patient discussion for primary care providers. Jun 1, 2022. ... Daily science news on research developments and the latest scientific innovations.
Abstract. Osteoporosis (OP) is a chronic bone disease characterized by aberrant microstructure and macrostructure of bone, leading to reduced bone mass and increased risk of fragile fractures. Anti-resorptive drugs, especially, bisphosphonates, are currently the treatment of choice in most developing countrie ….
"This opens up a new area of study to allow for clinical application to prevent or reverse osteoporosis," said Maria Esteban Lopez, who worked with Agoulnik on this research as an FIU biomedical sciences Ph.D. candidate. "This was an exciting opportunity.
Current research strategies to find new target factors for osteoporosis treatment involve investigating the genomics, proteomics, epigenetics, and metabolomics of human samples . To date, a large number of osteoporosis-associated genome-wide association studies (GWAS) have been carried out to identify the genetic risks of osteoporosis [ 118 ].
20th Annual Osteoporosis: New Insights in Research, Diagnosis, and Clinical Care *Live Stream* July 13, 2023. MEP23001 Osteoporosis 2022 *Live Stream* August 4, 2022. MEP22001 Osteoporosis 2021: New Insights in Research, Diagnosis and Clinical Care *Live Stream* July 15, 2021
Since the development of denosumab, new drugs for osteoporosis treatment have been discovered by careful analysis of rare bone diseases and bone cell biology, particularly subcellular assessment of the osteoclast—underlining the importance of translational research. 39 Thus, the two human bone phenotypes of sclerosteosis 40 and van Buchem's ...
Osteoporosis, a common bone disease characterized by thinning of bone tissue, affects one in five Americen women over the age of 50. In healthy individuals, bone is continuously formed and reabsorbed by the body. This balance is upset in osteoporotic patients. In their systems, stem cells that normally differentiate into bone-forming cells seem ...
Treatment with 10 mg of alendronate daily for 10 years produced mean increases in bone mineral density of 13.7 percent at the lumbar spine (95 percent confidence interval, 12.0 to 15.5 percent ...
The results were recently published in the Journal of Bone and Mineral Research. Osteoporosis causes 120,000 bone fractures in Sweden every year - including 18,000 hip fractures - and half of ...
The good news is that osteoporosis need not occur. Based on a study of the largest twin registry in the world, less than 30 percent of osteoporotic fracture risk is heritable. Researchers concluded that "fracture-prevention efforts at older ages should be focused on lifestyle habits.". This is consistent with the enormous variation in hip ...
The Journal of Bone and Mineral Research provides a diverse, highly impactful research with the latest insights into musculoskeletal system and mineral metabolism. It is worth noting that New England Journal of Medicine, with its impact factor of 74.699, has also published many studies on osteoporosis rehabilitation. ... Research on ...
New research has found a link between eating eggs and improved bone density, which indicates a lower risk of osteoporosis. Egg consumption could improve the body's production of a specific ...
Treatment. Treatments for osteoporosis usually include: medications to strengthen a person's bones, such as bisphosphonates and biologics. fall prevention methods to help prevent fractures ...
Clinicians are being encouraged to refer patients with osteoporosis to a heavy lifting exercise program to improve bone health and reduce the risk of fragility fractures which can occur from minimal trauma such as a fall. Those fractures can dramatically reduce quality of life, lead to a loss of independence, and increase risk of an earlier death.
Traditional pathophysiological concepts of osteoporosis focused on endocrine mechanisms such as estrogen or vitamin D deficiency as well as secondary hyperparathyroidism. However, research over the last decades provided exiting new insights into mechanisms contributing to the onset of osteoporosis, which go far beyond this.
In the realm of osteoporosis, Pfizer offers key drugs like Forteo, Xeljanz, and Duavee, catering to the treatment and prevention of bone loss. Notable for breakthroughs like Viagra and Prevnar 13, Pfizer continues its pioneering research, collaborating and conducting clinical trials to address unmet medical needs in osteoporosis management.
This research sheds new light on the intricate connections between NDDs and fractures, offering valuable insights into potential risk factors and causal links. The bidirectional causal relationships between ADHD, ASD, and specific fractures highlight the need for comprehensive clinical approaches that consider both NDDs and physical well-being.
Abstract. Approximately 10 million men and women in the U.S. have osteoporosis, 1 a metabolic bone disease characterized by low bone density and deterioration of bone architecture that increase the risk of fractures. 2 Osteoporosis-related fractures can increase pain, disability, nursing home placement, total health care costs, and mortality. 3 ...