- U.S. Department of Health & Human Services
- National Institutes of Health

En Español | Site Map | Staff Directory | Contact Us
- Science Education
- Science Topics
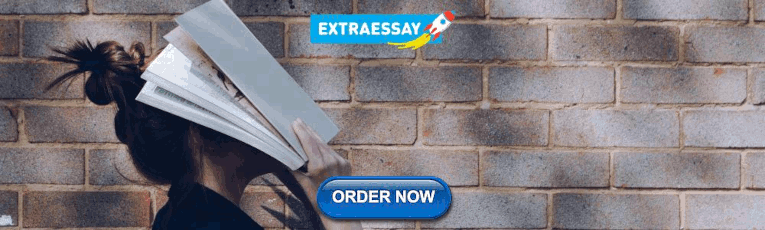
What is medical ultrasound?
How does it work, what is ultrasound used for, are there risks, what are examples of nibib-funded projects using ultrasound.
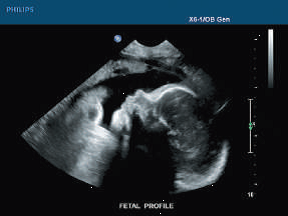
Medical ultrasound falls into two distinct categories: diagnostic and therapeutic.
Diagnostic ultrasound can be further sub-divided into anatomical and functional ultrasound. Anatomical ultrasound produces images of internal organs or other structures. Functional ultrasound combines information such as the movement and velocity of tissue or blood, softness or hardness of tissue, and other physical characteristics, with anatomical images to create “information maps.” These maps help doctors visualize changes/differences in function within a structure or organ.
Therapeutic ultrasound also uses sound waves above the range of human hearing but does not produce images. Its purpose is to interact with tissues in the body such that they are either modified or destroyed. Among the modifications possible are: moving or pushing tissue, heating tissue, dissolving blood clots, or delivering drugs to specific locations in the body. These destructive, or ablative, functions are made possible by use of very high-intensity beams that can destroy diseased or abnormal tissues such as tumors. The advantage of using ultrasound therapies is that, in most cases, they are non-invasive. No incisions or cuts need to be made to the skin, leaving no wounds or scars.
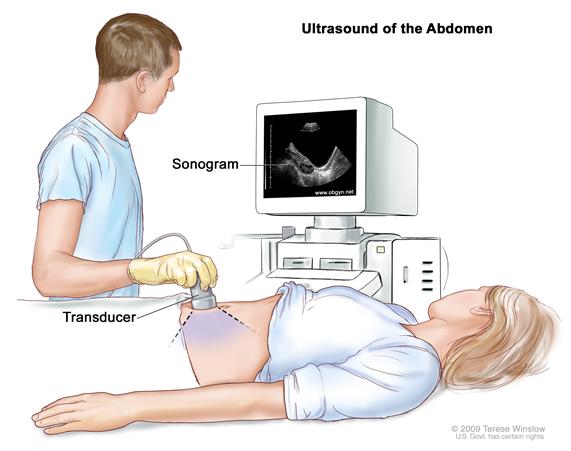
Ultrasound waves are produced by a transducer, which can both emit ultrasound waves, as well as detect the ultrasound echoes reflected back. In most cases, the active elements in ultrasound transducers are made of special ceramic crystal materials called piezoelectrics. These materials are able to produce sound waves when an electric field is applied to them, but can also work in reverse, producing an electric field when a sound wave hits them. When used in an ultrasound scanner, the transducer sends out a beam of sound waves into the body. The sound waves are reflected back to the transducer by boundaries between tissues in the path of the beam (e.g. the boundary between fluid and soft tissue or tissue and bone). When these echoes hit the transducer, they generate electrical signals that are sent to the ultrasound scanner. Using the speed of sound and the time of each echo’s return, the scanner calculates the distance from the transducer to the tissue boundary. These distances are then used to generate two-dimensional images of tissues and organs.
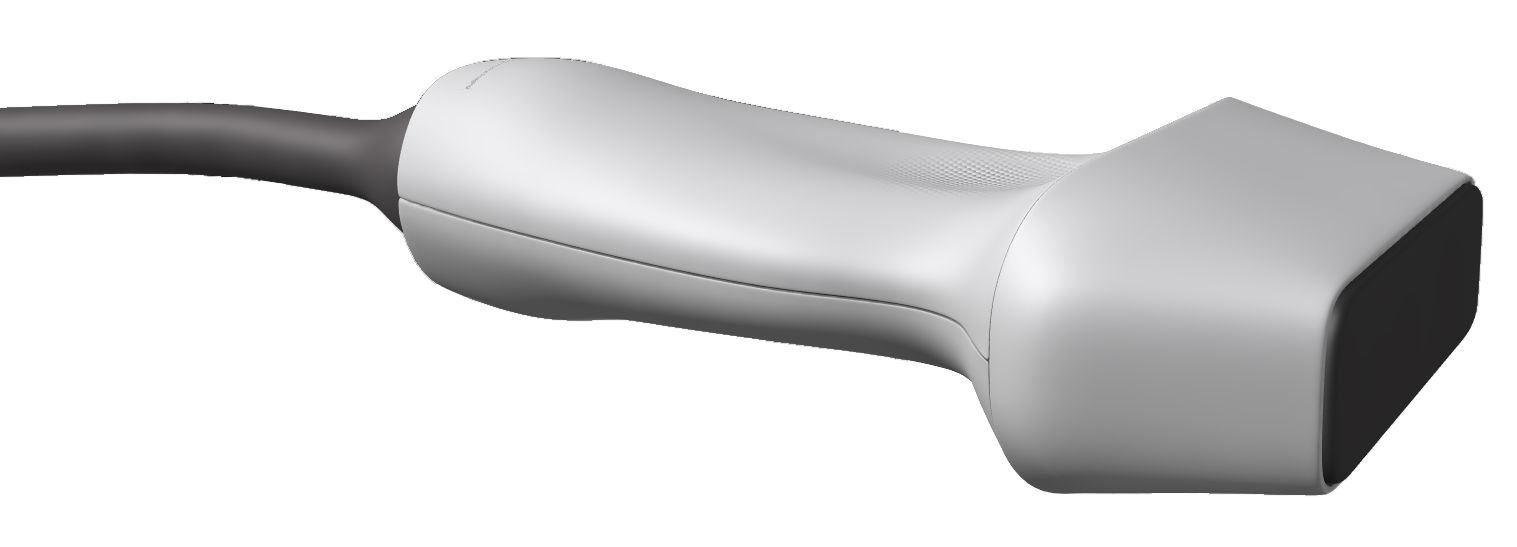
During an ultrasound exam, the technician will apply a gel to the skin. This keeps air pockets from forming between the transducer and the skin, which can block ultrasound waves from passing into the body.
Click here to watch a short video about how ultrasound works.
Diagnostic ultrasound. Diagnostic ultrasound is able to non-invasively image internal organs within the body. However, it is not good for imaging bones or any tissues that contain air, like the lungs. Under some conditions, ultrasound can image bones (such as in a fetus or in small babies) or the lungs and lining around the lungs, when they are filled or partially filled with fluid. One of the most common uses of ultrasound is during pregnancy, to monitor the growth and development of the fetus, but there are many other uses, including imaging the heart, blood vessels, eyes, thyroid, brain, breast, abdominal organs, skin, and muscles. Ultrasound images are displayed in either 2D, 3D, or 4D (which is 3D in motion).
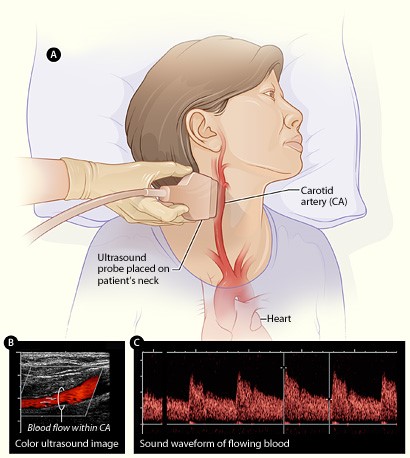
Functional ultrasound. Functional ultrasound applications include Doppler and color Doppler ultrasound for measuring and visualizing blood flow in vessels within the body or in the heart. It can also measure the speed of the blood flow and direction of movement. This is done using color-coded maps called color Doppler imaging. Doppler ultrasound is commonly used to determine whether plaque build-up inside the carotid arteries is blocking blood flow to the brain.
Another functional form of ultrasound is elastography, a method for measuring and displaying the relative stiffness of tissues, which can be used to differentiate tumors from healthy tissue. This information can be displayed as either color-coded maps of the relative stiffness; black-and white maps that display high-contrast images of tumors compared with anatomical images; or color-coded maps that are overlayed on the anatomical image. Elastography can be used to test for liver fibrosis, a condition in which excessive scar tissue builds up in the liver due to inflammation.
Ultrasound is also an important method for imaging interventions in the body. For example, ultrasound-guided needle biopsy helps physicians see the position of a needle while it is being guided to a selected target, such as a mass or a tumor in the breast. Also, ultrasound is used for real-time imaging of the location of the tip of a catheter as it is inserted in a blood vessel and guided along the length of the vessel. It can also be used for minimally invasive surgery to guide the surgeon with real-time images of the inside of the body.
Therapeutic or interventional ultrasound. Therapeutic ultrasound produces high levels of acoustic output that can be focused on specific targets for the purpose of heating, ablating, or breaking up tissue. One type of therapeutic ultrasound uses high-intensity beams of sound that are highly targeted, and is called High Intensity Focused Ultrasound (HIFU). HIFU is being investigated as a method for modifying or destroying diseased or abnormal tissues inside the body (e.g. tumors) without having to open or tear the skin or cause damage to the surrounding tissue. Either ultrasound or MRI is used to identify and target the tissue to be treated, guide and control the treatment in real time, and confirm the effectiveness of the treatment. HIFU is currently FDA approved for the treatment of uterine fibroids, to alleviate pain from bone metastases, and most recently for the ablation of prostate tissue. HIFU is also being investigated as a way to close wounds and stop bleeding, to break up clots in blood vessels, and to temporarily open the blood brain barrier so that medications can pass through.
Diagnostic ultrasound is generally regarded as safe and does not produce ionizing radiation like that produced by x-rays. Still, ultrasound is capable of producing some biological effects in the body under specific settings and conditions. For this reason, the FDA requires that diagnostic ultrasound devices operate within acceptable limits. The FDA, as well as many professional societies, discourage the casual use of ultrasound (e.g. for keepsake videos) and recommend that it be used only when there is a true medical need.
The following are examples of current research projects funded by NIBIB that are developing new applications of ultrasound that are already in use or that will be in use in the future:
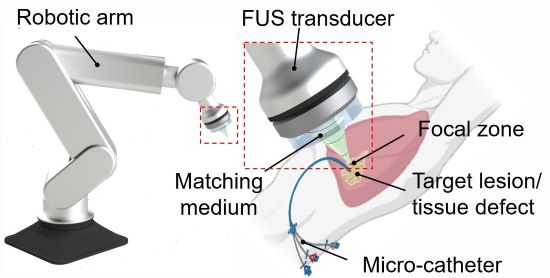
3D printing through the skin : Researchers at Duke University have developed a method to 3D print biocompatible structures through thick, multi-layered tissues. The approach entails using focused ultrasound to solidify a special ink that has been injected into the body to repair bone or repair soft tissues, for example. Initial experiments in animal tissue suggest the method could turn highly invasive surgical procedures into safer, less invasive ones. (Image on left courtesy of Junjie Yao (Duke University) and Yu Shrike Zhang (Harvard Medical School and Brigham and Women’s Hospital)).
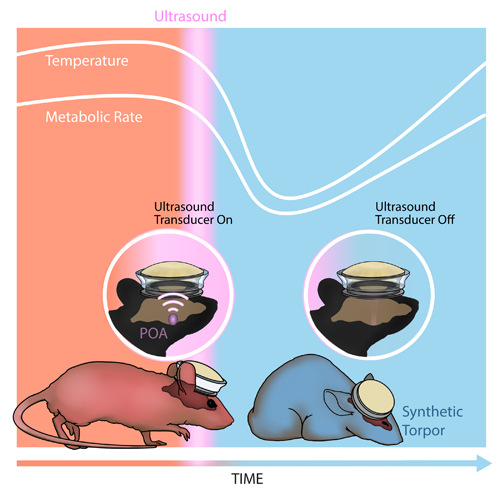
Inducing a hibernation-like state : Researchers at Washington University in St. Louis used ultrasound waves directed into the brain to lower the body temperature and metabolic rates of mice, inducing a hibernation-like state, called torpor. The researchers replicated some of these results in rats, which, like humans, don’t naturally enter torpor. Inducing torpor could help minimize damage from stroke or heart attack and buy precious time for patients in critical care. (Image on right courtesy of Yang et al./Washington University in St. Louis).
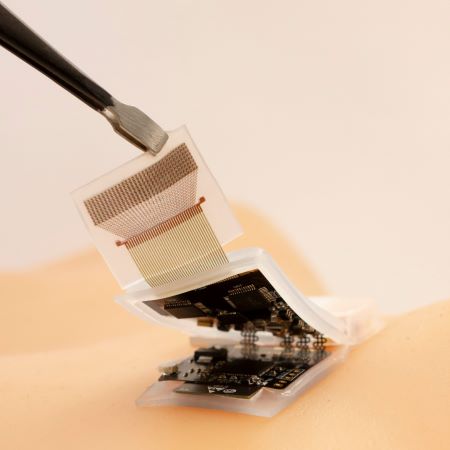
High-quality imaging at home : Brigham and Women’s Hospital researchers compared ultrasound scans acquired by experts with those taken by inexperienced volunteers, finding little difference in the image quality of the two groups. The unconventional approach of having patients take ultrasound images of themselves at home and share them with healthcare professionals could allow for remote monitoring and reduce the need for hospitalization. (Image on right courtesy of Duggan et al./Brigham and Women's Hospital).
Reviewed December 2023

Explore More
Heath Topics
- Breast Cancer
- Cardiovascular Disease
- Digestive Diseases
- Heart Disease
- Musculoskeletal Disease
- Reproductive Health
- Women's Health
Research Topics
- Optical Imaging
- Ultrasound (US) diagnostic
- Ultrasound (US) therapy
PROGRAM AREAS
- Ultrasound: Diagnostic and Interventional
Inside NIBIB
- Director's Corner
- Funding Policies
- NIBIB Fact Sheets
- Press Releases

An official website of the United States government
The .gov means it’s official. Federal government websites often end in .gov or .mil. Before sharing sensitive information, make sure you’re on a federal government site.
The site is secure. The https:// ensures that you are connecting to the official website and that any information you provide is encrypted and transmitted securely.
- Publications
- Account settings
Preview improvements coming to the PMC website in October 2024. Learn More or Try it out now .
- Advanced Search
- Journal List
- HHS Author Manuscripts

Ultrasound in Radiology: from Anatomic, Functional, Molecular Imaging to Drug Delivery and Image-Guided Therapy
Alexander l. klibanov.
1 Cardiovascular Division; Robert M. Berne Cardiovascular Research Center, University of Virginia School of Medicine, Charlottesville VA 22908
2 Department of Biomedical Engineering, University of Virginia, Charlottesville VA 22908
John A. Hossack
During the past decade, ultrasound has expanded medical imaging well beyond the “traditional” radiology setting - a combination of portability, low cost and ease of use makes ultrasound imaging an indispensable tool for radiologists as well as for other medical professionals who need to obtain imaging diagnosis or guide a therapeutic intervention quickly and efficiently. Ultrasound combines excellent ability for deep penetration into soft tissues with very good spatial resolution, with only a few exceptions (i.e. those involving overlying bone or gas). Real-time imaging (up to hundreds and thousands frames per second) enables guidance of therapeutic procedures and biopsies; characterization of the mechanical properties of the tissues greatly aids with the accuracy of the procedures. The ability of ultrasound to deposit energy locally brings about the potential for localized intervention encompassing: tissue ablation, enhancing penetration through the natural barriers to drug delivery in the body and triggering drug release from carrier micro- and nanoparticles. The use of microbubble contrast agents brings the ability to monitor and quantify tissue perfusion, and microbubble targeting with ligand-decorated microbubbles brings the ability to obtain molecular biomarker information, i.e., ultrasound molecular imaging. Overall, ultrasound has become the most widely used imaging modality in modern medicine; it will continue to grow and expand.
Introduction
Over the decade 2000–2011, the annual global per-procedure usage of X-Ray Computed Tomography (CT) and Magnetic Resonance Imaging (MRI) doubled [ 1 ]. Meanwhile, the number of ultrasound imaging procedures increased more than tenfold [ 1 ]. It is clear to practicing radiologists that this increase in ultrasound imaging usage has not occurred in the traditional medical imaging settings - radiology and cardiology departments in hospitals. In this review, we will examine recent developments and potential future directions in ultrasound imaging instrumentation that will explain the rapid changes in the practice of medical ultrasound imaging.
Similar to the other imaging modalities, ultrasound is approaching limits imposed by the underlying physics (e.g. wavelength) and regulatory tolerance for patient exposure to any form of energy transmitted into the body. Therefore, it becomes interesting to speculate how the different modalities may evolve in the coming years in the face of fundamental physics-origin limitations. As in other imaging modalities, application of contrast agents may assist in overcoming some of the limitations, and brings ultrasound imaging to the areas where its application was not feasible earlier, such as molecular imaging, therapy and drug delivery. In a short manuscript format it is not possible to provide an exhaustive review of literature; we provide examples of the specific trends of highest interest.
Fragmentation of the Practice of Ultrasound Imaging
Until the early 2000s, ultrasound imaging was dominated by cart-based systems priced in the ∼$100–200K range. Physicians, or specialized imaging technicians, in hospital radiology and cardiology departments operated these instruments. More recently, ultrasound imaging has proliferated in the form of hand-held and laptop-size instruments that are finding use in a range of settings beyond conventional large hospital imaging-focused departments. By extension, the reduced size, reduced cost and perceived reduced training requirement of these smaller devices make them feasible options for overseas and underserved communities, as well as ambulance and house call scenarios. This change has been enabled by the Moore’s Law [ 2 ] related improvement in the performance of electronics. Specialized, single purpose, signal processing hardware has been replaced by systems in which software controls a range of functions implemented on powerful, versatile, digital processors with very high data bandwidths [ 3 , 4 ]. Today’s hand carried instruments can essentially achieve functionality and imaging performance similar to the earlier cart-based instruments [ 5 ]. Small-scale instruments are finding uses that include guiding vascular access [ 6 ], emergency room assessment for conditions such as chest pain [ 7 ], detecting blood pooling or foreign object identification / removal [ 8 ]. Significantly, these instruments are primarily operated by healthcare workers for whom imaging is not their primary clinical specialty. Medical ultrasound has been taken to the International Space Station [ 9 ] and to the battlefield [ 10 ]. Remote real-time image viewing (i.e. “telemedicine”) has been demonstrated in both scenarios [ 9 , 10 ].
Figure 1 illustrates two recently commercialized handheld ultrasound scanners. It is clear that we are approaching a scenario in which size of the device is not limited by electronics but by the need for a transducer of a specific aperture according to the clinical necessity and the requirement for a usefully dimensioned image display. Currently, battery dimension and charge duration are also significant considerations but progress in battery technology and processor efficiency provide optimism for continued improvement towards a widespread use of handheld and laptop-based ultrasound.

Portable ultrasound equipment. (Left) “SonicWindow” Handheld C-scan device (Reprinted with permission, image courtesy of Analogic, Copyright, 2014) (Right) “V-Scan” Handheld B-scan device (Reprinted with permission, image courtesy of GE Healthcare, Copyright 2013)
In essence, the Moore’s Law contribution to the field is greatest in areas where massive computational processing requirements are most relevant. Until recently, many of signal / image processing algorithms that existed only in research laboratories could not be translated to widespread clinical usage. Now, however, progressively more complex signal and image processing tasks come within the realm of practicality and viable cost.
Each imaging modality is capable of anatomic, functional and molecular imaging – with varying degrees of performance and limitation. Anatomic imaging remains the dominant application even as the increasing values of functional and molecular imaging become more evident. Unfortunately, ultrasound imaging does not reliably perform well at anatomic imaging. Ultrasound relies on weak reflections and low rates of beam refraction. Generally, in soft tissue, the weak reflection requirement is met. The weak reflection requirement is not met when there is an interface involving materials possessing significantly different acoustic impedances. In ultrasound, bones and air volumes (in lungs, esophagus, and the gastrointestinal tract) present challenges and give rise to limited “acoustic windows” in some applications. For example, cardiac imaging relies on a relatively small number of acoustic windows between or below the ribs. The weak reflection requirement is not met in some other scenarios giving rise to either, or both, acoustic shadowing and acoustic reverberation. In the former, ultrasound does not reach underlying tissue regions and no echo signal is received resulting in a black region. A typical example involves the inability to “see” below an overlying rib. Reverberation typically occurs between overlying layers of muscle and fat and causes multi-path reflection [ 11 ]. This is projected as a phantom, static, echo haze-like image appearing at a greater depth than the actual source of the initial reflection. In radiology applications, the “haze” obscures the tissue of interest to varying degrees contributing to reduced image contrast and less reliable diagnosis.
This phenomenon occurs in cardiac imaging and may cause a static haze over the superficial moving cardiac muscle or appears, for example, as a static object within a heart chamber. Another important class of ultrasound artifact frequently encountered relates to “phase aberration”. Ultrasound beam formation relies on assumed tissue velocity. Since fat possesses a significantly lower sound speed (1478m/s) than muscle tissue (1547 m/s) [ 12 ], a layer of non-uniformly thick superficial fat, or distributed fat, can give rise to refractive errors in the beam focusing process. This results in a degraded beam resolution that has impact on both image spatial (“detail”) resolution and image contrast quality. These artifact mechanisms give rise to a variety of opportunities in research and point towards areas of probable progress in the coming decade.
Phase aberration correction
Phase aberration has been extensively studied since the late 1980s [ 13 ]. Flax and O’Donnell’s work represented a major early contribution towards a practical solution in that it was the first to not require a “beacon” or ideal point source. During the 1990s, efforts were made to implement phase aberration correction on clinical scanners but cost and technical complexity impeded progress. Additionally, it was realized that ideal phase aberration correction requires aberration delay correction in both elevational and azimuthal dimensions on an array surface [ 14 , 15 ]. Building from the concept of receive direction phase distortion, Liu and Waag proposed a “back propagation” approach in which compensatory delay corrections are made on the transmit direction [ 16 ]. A closely related technique involves the use of “time reversal” which also uses received phase errors to guide subsequent transmitted waves [ 17 ]. This technique works optimally with a well defined point source or point scatterer. More recently, Pernot [ 18 ] proposed the generation of cavitation bubbles to provide a nearly ideal source for the time reversal approach. Considerable work over many years has resulted in progressively more sophisticated algorithms with improved performance versus the earlier element signal cross correlation approaches. These include development of a new generalized coherence factor (GCF) [ 19 ] in which the lower frequencies correspond to the phase aligned components of received echo data, and the higher frequencies correspond to the poorly phase aligned echo signals. Additionally, new applications are being proposed that include, for example, phase aberration correction to improve the performance of therapeutic transcranial focused ultrasound [ 20 – 23 ].
“Tissue Harmonic Imaging” (THI)
Nonlinear ultrasonic propagation effects have been studied for several decades. During propagation at high pressure levels, local sound speed changes between compressional and rarefactional phases giving rise to distortion of the wavefront. This progressive increase in signal distortion gives rise to the presence of harmonics of the transmitted signal and these harmonics can be isolated for imaging purposes. Christopher, in 1997 [ 24 ], focused attention on the potential impact of imaging using the tissue harmonic signal and, in particular, it was noted that the nonlinear tissue signal would have reduced sidelobes and improved performance when propagating through typical abdominal wall sections. Within a few months following this paper, all major ultrasound imaging equipment vendors offered some variant on tissue harmonic imaging. In current imaging usage, THI, rather than conventional fundamental mode imaging, is frequently the default operating mode [ 25 ]. Initially, it had been assumed that the low signal level of the nonlinear signal would be objectionable but practical experience indicates that the level of performance achieved in practical settings vastly outweighs a relatively modest impact on maximum imaging depth. One minor drawback of tissue harmonic imaging is that it has motivated widespread use of higher peak power intensity in order to generate the greatest nonlinear signal and to minimize the impact of reduced SNR. Tissue harmonic imaging has proven to be one of the major contributions towards improved clinical B-Mode ultrasound quality [ 26 , 27 ]. Significantly, tissue harmonic imaging made the greatest clinical impact in imaging through the abdominal wall of patients with thicker abdominal walls – i.e. it has made the most impact in the population most likely to benefit from diagnostic ultrasound. It is only in recent years that comprehensive simulations, enabled by high power computation, have elucidated the underlying roles of phase aberration and nonlinear signal generation in the remarkable improvements in image quality observed in clinical THI imaging [ 28 ].
Model-based segmentation and imaging
Frequently, in radiology it is desirable to assess the shape or volume of a particular tissue region. In the case of objects with well-constrained shapes it becomes feasible to eliminate imaging deficiencies (overlying noise, speckle effects, etc.) by fitting a model to the acquired data and presenting to the user a model closely fitted to the acquired image data. For example, a tumor mass or the left ventricle of the heart is known to possess a convex shape that can be fitted and presented to the user as a perfectly “clean” representation of the ventricle – with a seemingly realistic smooth surface and no overlying clutter. Once the model is determined, clinically important linear, area and volume dimensions may be reliably generated. The primary limitation of any model-based fitting approach is that if the model is poorly selected then the method will fail to obtain an accurate fit to the actual available image data. In cancer radiology, there is interest in following the serial evolution of a tumor to assess growth or regression. The most reliable measure of tumor size is a determination of volume (3D) rather than a linear dimension (1D) or maximal cross-sectional area (2D). These two latter measurements are susceptible to error, due to incorrect slice plane selection and both implicitly rely on simplistic geometric assumptions relating linear dimension, or area, to volume. Unfortunately, tumors and other masses can take a number of shapes making model fitting challenging. One of the most versatile fitting models in image processing involves “snakes” or “active contours” [ 29 ]. Although originally developed as a 2D model, the snake is readily extensible to 3D [ 30 ]. The snake formulation involves the use of “internal energy” and “external energy” [ 29 ]. “Internal energy”, which can be user refined, forces the fitted closed line (or volume) shape to adopt a controlled degree of smoothness. (Technically, this involves a constraint on the second derivative along the resulting snakes contour.) In the case of a noisy ultrasound image, this parameter is particularly important. “External energy” is the parameter that forces the fitted line to best conform to the underlying image data. This is most frequently derived from the image gradient (i.e. image feature edges). Since the purpose is to find the underlying feature gradient, uncontaminated by ultrasound image speckle, snake processing on ultrasound data frequency involves a speckle reducing pre-processing step [ 31 , 32 ]. There are a number of frequently encountered speckle reducing algorithms [ 33 – 38 ]. Among these algorithms, Yu’s Speckle Reducing Anisotropic Diffusion (SRAD) is the most commonly encountered and has been extended to 3D [ 39 ]. Several examples of the use of snakes in ultrasound feature segmentation exist for both 2D [ 40 ] and 3D [ 41 , 42 ].
Artifact reduction
One of ultrasound’s primary weaknesses as an anatomic imaging modality, in comparison with MRI and CT, is its susceptibility to artifacts. This motivates a range of efforts to solve ultrasound’s artifact problems. Fortunately, evolving processing speed enables the clinical realization of progressively more complex algorithms. Except in the case of tissue harmonic imaging, in which the nonlinear second harmonic of the transmitted fundamental is isolated (i.e. filtered) for image formation, the challenge in ultrasound image data processing is that desired signals and artifacts (e.g. reverberations) cannot be isolated by simple frequency domain filtering.
Frequently, the contrast qualities of an image can be improved by spatial compounding in which a common tissue region is viewed from independently oriented transmit beam angles [ 43 ]. Spatial compounding has been shown to reduce speckle, clutter and improve image qualities and the ability to differentiate tissues [ 43 ]. In particular, it has proven successful in breast ultrasound: increased conspicuity of lesions, improved margin delineation and clearer cystic imaging have been reported [ 44 ]. A limitation, however, of transmit beam spatial compounding is that the aperture must be divided to yield independent apertures and this results in reduced resolution on a per aperture basis.
In the case of moving tissue one can, in principle, differentiate the desired moving signal from an overlying static haze artifact [ 45 – 47 ]. While most of these algorithms are computationally intensive, they have the potential for widespread incorporation into the future generation of clinical scanners. This class of artifact reduction has more utility in cardiology than in radiology.
Quantification – tissue tracking
Within the past decade regional tissue tracking (“speckle tracking”) has rapidly evolved from a research topic to a commonly implemented clinical ultrasound feature. Although most applications of speckle tracking are in cardiology [ 48 – 50 ], there are a number of radiology applications. Golemati et al [ 51 ] discussed the utility of speckle tracking for assessing elastic properties of the arterial wall and plaque in carotid arteries.
Advances in Doppler
Traditional Color Doppler processing is limited to detecting the 1D component of motion aligned with the ultrasound beam axis. Kasai’s [ 52 ] 1D autocorrelation-based method formed the basis of the vast majority of early clinical scanner implementations for Color Doppler. Kasai’s approach is computationally very efficient but involves a narrow signal bandwidth assumption. Kasai’s approach was largely supplanted by Loupas’ [ 53 ] 2D method which estimates the Doppler value using explicit estimates of both mean Doppler frequency and mean RF frequency at each range segment. It is also possible to estimate velocities using time domain cross-correlation [ 54 ] but this method is less frequently encountered in clinical implementations. However, all these early methods extract only the Doppler component aligned with the ultrasound beam axis. Given that vessels are frequently parallel to the skin surface (e.g. carotid artery), this represents a major limitation. In principle, this problem can be addressed using speckle tracking [ 55 ] but this typically requires high signal to noise ratio and, thus, is limited to shallow vessels like the carotid artery. Speckle tracking is also a relatively computationally intensive process. Another solution for enabling Doppler detection of transverse motion is to introduce oscillation into the transverse direction [ 56 – 58 ]. Using this approach, true “vector Doppler” (i.e. 2D Doppler) is achievable. Synthetic aperture-based approaches have also been proposed that transmit across all directions simultaneously and consequently can form images across the entire field of view at once [ 59 ]. By stepping the aperture source element across the array aperture, and combining the results from multiple transmit events, a high resolution wide-field Doppler image is acquired. More recently, there has been growth in the area of plane wave Doppler processing [ 60 , 61 ]. Multiple acquisitions are obtained from multiple angles and these are combined. This approach provides for very high frame rates (>1000 fps vs. 10’s fps in conventional Color Doppler) across wide fields of view (see Figure 2 ). Significantly, Bercoff notes that real-time implementation of this Doppler processing places very high demands on processor speed and data bandwidth [ 61 ]. Bercoff’s work was implemented on the Aixplorer ultrasound system (SuperSonic Imagine, France). This is the first clinical system with the required processing capability for this new Doppler method and suggests where the “high end” of clinical ultrasound is evolving. Since kHz Color Doppler has only recently become available, we are only now learning about its value in diagnoses. It is also noteworthy that these frame rates place them well beyond the foreseeable capabilities of competing imaging modalities even as frame improvements in competing modalities are enabled by significant technical advances.

Selected frames from a cardiac cycle obtained with using ultrafast compound Doppler. (a) Average flow in the artery indicating the selected frames. (b) Before the opening of the aortic valve, there is a minimal laminar flow. (c) and (d) Acceleration of the flow. (e) Inversion of the parabolic profile in the deceleration. (f) Local turbulence is present and propagates in the artery. (g) and (h) Laminar flows in diastole. Reprinted with permission from [ 61 ], Copyright, 2011, IEEE.
Catheter-based ultrasound
Intravascular Ultrasound (IVUS) has been available clinically for more than two decades. Although the majority of IVUS applications are in cardiology, there are a number of uses within interventional radiology. IVUS technology generally divides into those based on mechanically scanned single transducer element and those comprising a solid-state circumferential array [ 62 ]. IVUS provides cross-sectional vessel anatomic structural information that is not obtained using only X-Ray angiography – which gathers information from a projection across the vessel lumen. IVUS also provides greater imaging depth than Optical Coherence Tomography (OCT); the latter is limited to ∼1 mm [ 63 , 64 ]. IVUS can provide a range of functional information about the vessel wall health and function [ 63 ], yet OCT is frequently presented as a technology that may potentially supplant IVUS based on its superior spatial resolution. Recently, there has been significant progress in the area of pairing IVUS and photoacoustic imaging [ 65 – 68 ]. IVUS can provide excellent anatomic information and some functional information. Photoacoustic imaging provides superior information on vessel wall composition [ 66 , 67 ] and can perform molecular imaging using appropriately targeted light absorbing nanoparticles [ 69 ] (see below).
Elastography
Early versions of elastography primarily relied upon an external application of force during which tissue motion was tracked in using phase sensitive approaches applied to the beamformed radio frequency (RF) line data [ 70 , 71 ]. Over the years, many improvements have been proposed to the underlying algorithms to improve precision and accuracy [ 72 – 74 ][ 75 – 77 ]. The method has found application in a range of settings that include: breast [ 78 , 79 ], prostate [ 80 ] and thyroid [ 81 ]. The approach has been scaled down and performed using IVUS to assess vessel wall elasticity [ 82 – 84 ]. A major contribution in the field of elasticity involved the realization that acoustic radiation force could be used to project the force from within the body at a precise location instead of relying on an external force that rapidly decays with depth and is susceptible to artifacts due to intervening inhomogeneities [ 85 – 87 ]. This version of elasticity imaging is now in clinical usage [ 88 ]. More recently, considerable interest has arisen in shear wave elasticity imaging. Since a shear wave propagates slowly in tissue, the wavelength is low, which improves spatial resolution. Among the various implementations, supersonic shear imaging [ 89 ] appears to be the most promising and has yielded very encouraging early results. In this approach, a supersonic wavefront is created by rapid sequencing of pulses through depth, taking advantage of the fact that the shear wave velocity is just a few m/s. The method also relies on an ultrasfast scanner capable of full field imaging in response to a single transmit burst – i.e. similar to the Aixplorer ultrasound system previously mentioned. Promising results involving characterizing breast lesions have been reported using this approach [ 90 ]. It would appear that full frame, very high frame rate, systems like the Aixplorer most closely suggest the direction in which the “high end” ultrasound imaging field is going. It is also probable that a number of signal processing techniques, yet to be invented, will be enabled by this versatile high performance architecture.
Photoacoustic imaging
Photoacoustic (or optoacoustic) imaging involves the use of short duration laser pulses to induce transient thermal expansions giving rise to emitted ultrasound pulses emanating from the point of light absorption. The received ultrasound pulses are processed in a manner analogous to conventional ultrasound receive signal processing. The receive path may involve either a mechanically scanned single element ultrasound transducer or a phased ultrasound array. Photoacoustic imaging has experienced an extraordinarily rapid rate of technical development in the past decade. Consequently, it should be viewed as a completely new imaging modality as opposed to a subset of ultrasound imaging. Photoacoustic imaging is discussed only briefly in this review. The reader is referred to the review article by Xu and Wang [ 91 ] for a more extensive discussion.
Anatomic ultrasound image contrast is a function of tissue mechanical properties, tissue interfaces and backscatter density. Image contrast in photoacoustic imaging is determined by local light absorption conditions and this will typically also vary with optical wavelength. Thus, it is feasible to image and differentiate between oxygenated and deoxygenated blood [ 91 ], differentiate lipid-dominant versus water-dominant tissue signals and detect other light-absorbing chomophores (endogenous and exogenous). Traditional optical imaging is sensitive to these parameters but possesses extremely limited penetration due to light scattering at any significant depth. Photoacoustic imaging is projected to contribute across a wide range of clinical areas that include: endoscopic imaging, mapping of metabolic rate of oxygen, melanoma, breast cancer, brain pathologies, and mapping of sentinel lymph nodes [ 92 ]. Photoacoustic tomography, using reconstruction principles similar to those used in Computed Tomography, has extended the technical frontier in terms of very high resolution photoacoustic imaging [ 93 – 95 ]. Using these approaches, small animal whole body photoacoustic imaging systems have become developed [ 96 ] and are available commercially [ 97 ].
Photoacoustic imaging divides broadly into two modes of operation. In acoustic resolution photoacoustics, it is not necessary for the input light to be focused to a single point. Because of rapid light scattering in tissue, in any event the light signal becomes defocused beyond approximately 1 mm of depth. In this scenario, photoacoustic imaging resolution is determined by the focusing performance of the receive ultrasound beamformation process. It is a common misperception to believe that light cannot penetrate deep into tissue. By choosing the correct light wavelength (i.e. using Near Infra-Red (NIR: ∼700 to ∼900 nm) [ 91 ], several cm of imaging depth is achievable. Additionally, photoacoustics has extensive applications in catheter-based applications where it can be paired with conventional IVUS [ 65 , 66 , 98 ]. Photoacoustics can also be paired with
Optical Coherence Tomography (OCT) [ 99 ].
Figure 3 , from Hu et al, [ 100 ] illustrates a range of resolutions and sources of contrast that include optical resolution photoacoustic microscopy of sO2 in a mouse ear, acoustic resolution photoacoustic microscopy of hemoglobin concentration in a human palm, photoacoustic CT of Methylene Blue concentration in a rat sentinel lymph node, photoacoustic CT of cerebral hemodynamic changes in response to whisker stimulation in a rat and Photoacoustic endoscopy of a rabbit esophagus and adjacent tissue.

Components of Photoacoustic Tomography, with representative in vivo images across multiple resolution scales (A) Optical Resolution Photoacoustic Microscopy of sO2 in a mouse ear. (B) Acoustic Resolution Photoacoustic Microscopy of normalized total hemoglobin concentration, [hemoglobin], in a human palm. (C) Linear-array Photoacoustic Computed Tomography of normalized Methylene Blue concentration, [dye], in a rat sentinel lymph node (SLN). (D) Circular-array Photoacoustic Computed Tomography of cerebral hemodynamic changes, Δ [hemoglobin], in response to one-sided whisker stimulation in a rat. (E) Photoacoustic endoscopy of a rabbit esophagus and adjacent internal organs, including the trachea and lung. UST, ultrasonic transducer. Reprinted with permission from [ 100 ]; Copyright, 1012, American Association for the Advancement of Science.
Contrast agents in ultrasound imaging
Contrast materials are applied in all imaging modalities, and ultrasound is not an exception. Early ideas of blood pool contrast ultrasound imaging (first discovered by serendipity) [ 101 ] come from the use of air bubbles generated in saline, serum albumin solutions or viscous X-ray contrast media. Unlike water (or most of biological tissues), gas bubbles are very compressible; thus, in response to the passage of the ultrasound wave as the cycles of positive and negative pressure, microbubbles rapidly compress and expand about their equilibrium (ambient) pressure setting, with the particle diameter variation reaching several fold [ 102 ]; movement of gas-liquid interface creates secondary pressure waves, i.e., ultrasound scattering. Luckily for this field, relatively small bubbles, with the diameter somewhat less than of a red blood cell, can scatter MHz ultrasound very efficiently, and can be detected by ultrasound imaging, with excellent sensitivity. A single microbubble, with a sub-picogram mass, can be observed at multi-cm depth, with abundant clinical imaging systems, in real time (at 20–30 frames/s or faster) [ 103 ]. Thus, the dose of the administered ultrasound contrast material can be in the single milligram or sub-milligram range, of which most material is either fully natural (e.g., human serum albumin in Optison microbubbles [ 104 ], or synthetic fully biocompatible, such as phospholipids, e.g., in Definity [ 105 ] and Sonovue microbubbles [ 106 ]). Non-microbubble ultrasound contrast agents were tested widely at the preclinical stage, but have not yet made it to the clinical application level, perhaps due to the larger required dose and lower acoustic backscatter.
Ultrasound contrast is used as a general radiology intravascular agent worldwide (so far the USA is an exception, where only cardiac ultrasound contrast imaging is approved in the clinic). Worldwide, several million ultrasound contrast exams take place every year; due to the low dose of the contrast material, serious side effects are infrequent; for the patients with kidney impairment, where X-ray contrast or Gd-based MRI contrast agents are undesirable, microbubble contrast exam may become the preferred option [ 107 ].
In order to observe microbubble particles in the bloodstream, contrast-specific detection schemes and pulse sequences have been implemented, with multi-pulse detection schemes being the most efficient. A combination of phase inversion (i.e., compression-first and rarefaction-first pulses) and power modulation (i.e., pulses with varying ultrasound transmit amplitude) provides the best sensitivity to contrast along with an excellent suppression of the background tissue signal [ 108 ]; see accompanying video of microbubbles influx into the tumor vasculature in a murine model. Contrast mode is often used in combination with regular grayscale B-mode imaging for anatomy positioning (see Figure 4 ). It is important that microbubble detection in the tissues can be achieved at low acoustic power levels, i.e., without destroying the microbubbles.

Ultrasound imaging of subcutaneneous tumor in a murine model. Top Left: B-mode grayscale imaging (anatomy). Top Right: contrast mode (Cadence CPS), prior to microbubble administration. Bottom Left: contrast mode (Cadence CPS), following microbubble administration (at peak, ∼5 sec following iv bolus). Bottom Right: contrast mode (Cadence CPS), following microbubble administration (at peak, ∼30 sec following iv bolus). Imaging performed with Sequoia 512 scanner equipped with 15L8 probe.
Real-time ultrasound contrast imaging capability is often used for characterization of cancerous nodules: after an intravenous bolus injection, tissue arrival time for normal tissues, benign and malignant tumors may differ significantly [ 109 ]. Modern imaging equipment has a color-coded arrival time routine, which allows a distinct presentation of the contrast arrival time differential between the tumor and surrounding tissues.
Destruction-replenishment as a tool for perfusion contrast imaging
In the 1990s, at the time when microbubble detection was not as sensitive, the most efficient way to monitor microbubble contrast in the bloodstream was to destroy them by higher acoustic pressure of ultrasound. Typically, with mechanical index (MI) in excess of 0.3, and up to 1.9 (as allowed for the diagnostic imaging scanners) destruction is achieved within just one imaging frame. Therefore, taking advantage of this targeted microbubble destruction in the interrogated volume with intermittent timed frame collection, Kaul et al [ 110 ] devised a tool to monitor myocardial perfusion, with the aim to observe perfusion defects in heart muscle following myocardial infarction. Intermittent imaging was performed in synchrony with heart pulses, triggered by EKG, typically at end-systole. Following microbubble infusion, when microbubbles concentration in the vasculature reached a constant level, ultrasound imaging was initiated and performed at every heart beat, then at every other heartbeat, then every third, fourth and fifth heartbeat. Microbubbles from outside of the insonated field (typically, a thin slice, 1–5 mm thick and up to 5 −15 cm long and wide) start to refill the vasculature, larger arteries first, followed by arterioles, capillaries, postcapillary venules and veins. It has been suggested that by timing the interval of ultrasound pulses, the fraction of the blood in the particular portion of the vasculature (e.g., capillaries) can be estimated [ 111 ].
Lately, with the advent of high-sensitivity multipulse detection schemes, ultrasound contrast imaging does not require microbubble destruction anymore. Therefore, after a single destructive pulse, replenishment of microbubbles into the interrogated volume can be monitored in real time, with the traditional clinical imaging equipment at 20–50 frames per second [ 112 ], (see Figure 5 and accompanying video) and with the most modern equipment at 10 3 Hz or even faster [ 113 , 114 ].

Contrast ultrasound imaging of tumor vasculature perfusion in destruction-replenishment mode in a subcutaneous murine tumor model. Top Left: microbubbles within the tumor vasculature after intravenous administration, prior to the destructive pulse. Top Center: immediately after 2 s destructive pulse. Top Right: 2 s after cessation of the destructive pulse. Bottom Right: 6 s after destructive pulse. Bottom Center: 10 s after destructive pulse. Bottom Right: 20 s after destructive pulse. Imaging performed with Sequoia 512 scanner equipped with 15L8 probe (7 MHz, MI 0.2 for imaging, MI 1.9 for destruction).
Overall, perfusion studies with microbubble contrast are now routinely used in the clinical setting worldwide (except USA as of now); they can provide blood flux information in the settings where Doppler imaging is not useful due to smallest size of the vessels in the tissue (e.g., in a transplanted skin flap [ 115 ]). An unfortunate limitation of this technique is in the inability of ultrasound at imaging frequencies to transit through the human skull without attenuation; thus, brain perfusion studies that are routinely performed with functional MRI cannot be performed with contrast ultrasound unless there is a burr hole present [ 116 ].
Molecular (Targeted) Contrast Ultrasound Imaging
Expanding the ability of ultrasound imaging to collect information on the biological processes at the molecular and cellular level requires the use of a specialized contrast agents, targeted microbubbles [ 117 ]. The general idea is traditional for targeted contrast imaging: a contrast particle is conjugated with the targeting ligand that possesses affinity towards the disease marker. The particles are administered in vivo (e.g., intravenously), circulate in the body, and accumulate in the area of disease.
As the typical mean size of microbubble contrast agents is several micrometers, these agents are unable to probe the receptors located outside of the vascular bed. Although nanobubble studies have been repeatedly reported in the literature over the past decade [ 118 , 119 ]), the acoustic backscatter and particle lifetime are both rather low and these agents have not approached practical application; therefore, ultrasound contrast imaging of leaky neovasculature (e.g., as in enhanced permeability and retention effect, EPR, [ 120 ]) is most likely going to be limited to the phase-shift liquid fluorocarbon nanodroplet formulations [ 121 ].
Current progress of molecular imaging with micrometer-sized targeted bubbles has been significant. It started with a model ultrasound imaging study in vitro, in petri dishes, avidin-biotin targeting [ 117 ], and progressed rapidly towards the use of cell cultures and antibody-mediated targeting [ 122 ], followed by in vivo studies. Several targets were investigated with significant detail: thrombi, markers of inflammation, and markers of angiogenesis.
The simplest targeted contrast agent is already in clinical use: Sonazoid (perflubutane) formulation is approved for liver imaging in Japan and South Korea [ 123 ]. Targeting specificity of this agent is based on its lipid shell composition, phosphatidylserine. This phospholipid is a natural marker of apoptosis and a powerful driver for the phagocytic uptake of apoptotic cells [ 124 , 125 ], cell fragments and other particles [ 126 ] by the cells of reticuloendothelial system (RES) and any other phagocytic cells, e.g., leukocytes, specifically, neutrophils. The latter cell is the first to adhere to vascular endothelium in the acute inflammatory response to ischemia-reperfusion injury, e.g., in an experimental myocardial infarction [ 127 ], or in the acute kidney injury scenario [ 128 ], which allows targeted microbubble ultrasound imaging.
More specific endothelial markers of interest to microbubble targeting include selectins (P- and E-) and integrins, such as VCAM-1 and ICAM-1, which are expressed on the surface of vascular endothelium in response to inflammatory stimuli. Microbubble targeting of these molecules is achieved either via antibody placement on the bubble shell [ 129 ] or the use of smaller molecules, such as peptides [ 130 ], nanobodies [ 131 ] or carbohydrates [ 132 ]. The latter molecule, sialyl Lewis X (or A), is present on the business end of a natural leukocyte membrane protein P-selectin glycoprotein ligand −1 (PSGL-1), a ligand for P- and E-selectin, so it is suitable for microbubble targeting to the sites of inflammation [ 133 , 134 ] and to activated platelets [ 135 ].
Another significant application area for microbubble targeting is tumor vasculature: specific markers of vascular endothelium in the areas of malignant tumors can be successfully imaged by ligand-carrying microbubbles. Initially an antibody against the tumor vasculature biomarker α v β 3 was applied [ 136 ], later followed by targeting with other ligand molecules, such as modified RGD peptides [ 137 ]. VEGF Receptor 2 is another important biomarker of the malignant tumor vasculature; this molecule is already a popular target for tumor detection with other imaging modalities [ 138 ] as well as with ultrasound molecular imaging, via microbubbles decorated with anti-VEGFR2 antibodies, [ 139 ], or single-chain VEGF [ 140 ], which has been shown to achieve selective accumulation of microbubbles in the tumor neovasculature in a murine model (see Figure 6 ). A synthetic heterodimeric peptide combination was discovered as a smaller molecule combination tool for VEGFR2 targeting [ 141 ]. The latter microbubble formulation, BR55, is in the ongoing Phase 1/2clinical trial, (see {"type":"clinical-trial","attrs":{"text":"NCT02142608","term_id":"NCT02142608"}} NCT02142608 ). Prior to this, an early-phase BR55 clinical trial for prostate cancer patients with scheduled radical prostatectomy compared VEGFR2 histology with targeted ultrasound imaging. This early study suggested co-location of the tumor nodules by both methods [ 142 ]. Molecular ultrasound imaging may be used to assist with image-guided biopsy (e.g., in the breast and prostate cancer setting) and targeted therapeutic interventions.

Ultrasound Molecular Imaging of VEGFR2 with scVEGF-decorated microbubbles. Ultrasound imaging of subcutaneous colon adenocarcinoma. A, B-mode US image of tumor tissue marked by dotted line. B, Contrast US image of nontargeted MB after 6-minute dwell time. C, Contrast US image illustrates higher pixel intensity because of adherent scVEGF-MB. Copyright, 2010, Lippincott, Williams and Wilkins, reprinted with permission from reference [ 140 ].
Overall, a combination of targeted contrast ultrasound agents with the wide availability of contrast imaging modalities on the ultrasound imaging equipment may result in the use of ultrasound molecular imaging for targeted diagnostics, image-guided biopsy and therapy.
Ultrasound in therapy: thermal and mechanical
Focused ultrasound has been suggested as a therapeutic modality decades ago [ 143 ], although wider clinical use of this approach started much later [ 144 ]. Induction of local hyperthermia by focused ultrasound is based on localized energy deposition. MRI can serve as a tool for precise temperature monitoring in the target tissue, although ultrasound imaging is used for ultrasound therapy guidance in the clinic widely outside of US. Maintaining tissue temperature of ∼45°C for 30–60 minutes (or much higher temperatures, for just a few seconds) is sufficient to kill the cells in the focal zone. Ultrasound of 0.6–1 MHz frequency is often used; from many cm away, a focal zone with the size and shape of a grain of rice can be formed using a focused transducer [ 145 ]. KW power of the therapeutic apparatus can achieve the desired temperature in the focal zone within seconds. Multi-element arrays (optimally, with thousands of elements) allow rapid electronic steering of the focal spot to accelerate the procedure and completely cover the desired treatment zone [ 146 ]. Approved indications include uterine fibroid therapy [ 147 ] and palliative treatment of bone metastases [ 148 ]. Lower frequency ultrasound (220 KHz, necessary for penetrating human skull without heating it significantly) is now being investigated as a tool for ultrasound therapy in the brain [ 149 ]. Focused ultrasound heating is now being tested as a non-invasive replacement of neurosurgical/electrode placement approach for treatment of essential tremor [ 150 ]. We can hope that tumor therapy will be successful in clinical trials in the bone metastasis setting (beyond palliation), as well as for treatment of brain tumors (e.g., {"type":"clinical-trial","attrs":{"text":"NCT01698437","term_id":"NCT01698437"}} NCT01698437 ) or prostate cancer (e.g., {"type":"clinical-trial","attrs":{"text":"NCT02265159","term_id":"NCT02265159"}} NCT02265159 ). Success of this non-invasive therapeutic modality is supported by the ability to focus ultrasound tightly and rapidly deep within the body (even through the skull). Limitations are also based on physical constraints: ultrasound energy is attenuated and absorbed by the bones (thus, brain treatment requires an additional CT or MRI study to adjust the ultrasound pulse sequences to compensate for the shape and thickness of the skull). Ultrasound cannot efficiently travel through the gas phase, so lung treatment can only be performed for liquid-filled lungs [ 151 ]. In some instances, ribcage obscures access to the target (e.g., certain areas of the liver). There have been reports that ribs in the way of ultrasound beam had been resected prior to the treatment with a large aperture single element transducer [ 152 ]. However, more appropriate would be to use multi-element array and adjust the transmit power for the elements which are obscured by the ribs [ 153 ].
Histotripsy implies high-power pulverization of the tissues: a water fountain generated on the air-water interface in the focal zone of an ultrasound transducer is recreated by cavitation in vivo within the therapeutic target tissues, if peak negative acoustic pressure reaches 10 MPa [ 154 ]. Following this treatment, a void in the biological tissue is created: target tissue (e.g., tumor nodule) is destroyed to subcellular level and liquefied [ 155 ].
Thrombolysis with ultrasound
Enhancing the rate of thrombolysis with ultrasound has been suggested more than a decade ago [ 156 , 157 ]. The idea is quite similar to tissue ablation, as described in the previous section; the acoustic energy applied for thrombolysis may be significantly lower, often within the limits of diagnostic ultrasound imaging. Ultrasound pressure wave provides mechanical action on the biological tissues (including the clot and surrounding blood). Liquid media streaming improves convection of the participants of the thrombolytic cascade in proximity and within the clot structure, resulting in thrombolysis acceleration. Presence of even small doses of thrombolytic agents and/or microbubbles (micro-foci of energy deposition and microstreaming) further accelerates thrombolysis. We can hope that ultrasound-assisted thrombolysis, if applied quickly (e.g., in an ambulance setting) will aid in reduction of the clot size, which in turn may help save brain tissue following stroke or myocardium following myocardial infarction [ 158 ]. Ultrasound can be applied non-invasively [ 159 ], or via a catheter [ 160 ].
Ultrasound-microbubble combination as a tool for targeted drug and gene delivery
Ultrasound has been investigated as a tool for microbubble-assisted drug delivery for almost two decades. Initially [ 161 ] model drugs were incorporated into the bubble shell. Later, tumor therapy in response to insonation was achieved in animal models [ 162 , 163 ] - but that was feasible mostly for rather hydrophobic drugs, e.g., paclitaxel. Plasmid DNA could be attached onto the bubble shell electrostatically, and ultrasound-assisted transfection enhancement was observed with such constructs [ 164 ]. Attachment of drug-loaded liposomes onto the surface of microbubbles allows ultrasound-triggered drug delivery capability: in response to microbubble insonation the drug is released from the liposome core [ 165 ]. This has been shown to work with widely used anticancer drugs, e.g., doxorubicin [ 166 ] and paclitaxel [ 167 ].
A combination approach, where existing drug is simply co-administered along with clinical grade approved microbubbles and focused ultrasound, will obviously get to clinical trials faster. In this approach, stable cavitation of microbubbles within the vasculature is used to transiently alter permeability of blood-brain barrier [ 168 ]. The disruption of the barrier is mild and transient (permeability enhancement ceases within hours). However, large items, such as liposomes [ 169 ] and other nanoparticles [ 170 ] can be delivered into the brain tissue as efficiently as smaller items, such as antibodies [ 171 ], other drugs [ 172 ], or even Gd-based MRI contrast agents [ 173 ] (See Figure 7 as an example of penetration enhancement of Gd-DTPA across blood-brain barrier in a rat model). This approach has already led to an exciting demonstration of glioma treatment in a rodent model (curative in a significant fraction of animals) with a simple combination of long-circulating doxorubicin liposomes (doxil) and clinical perflutren microbubbles [ 174 ]. Success of therapy can be explained by the ability of PEG-coated liposomes to stay in the bloodstream for many hours, recirculate through the insonated area vasculature and extravasate for many hours, as long as the drug remains in the bloodstream and the barrier remains open. Expansion of this technique towards clinical applications could be predicted, ranging from tumor therapy [ 174 ] to Alzheimer treatment [ 175 , 176 ].

Ultrasound-induced opening of blood brain barrier in a rat model. Decafluorobutane microbubbles (stabilized with DSPC and PEG Stearate) were injected intravenously, immediately followed by focused ultrasound treatment (IGT, 1 Hz, 20ms pulses, 1–2 min treatment duration) and intravenous administration of Gd-DTPA. MRI contrast extravasation and accumulation (white focal spots in the center of the image) observed minutes after ultrasound treatment and Gd-DTPA administration. Imaging performed at UVA Molecular Imaging Center (7T MRI Clinscan, Bruker/Siemens). Copyright, Max Wintermark, 2014, reprinted with permission.
Similar therapeutic approach can be applied in the situations other than the blood-brain barrier, to any endothelial lining in the vasculature that precludes entry of the drug into the diseased tissue. This approach has been applied to deliver particles of adeno-associated virus to the insonated myocardium [ 177 ], as well as for the treatment of pancreatic cancer in an orthotopic xenograft mouse model, by a combination of anticancer drug gemcitabine and Sonovue microbubbles [ 178 ]. The latter combination has demonstrated interesting data in a clinical trial setting, initially showing the suppression of tumor growth in response to intravenous administration of gemcitabine, immediately followed by injections of Sonovue microbubbles every 3.5 min and continuous insonation of the primary tumor with an ultrasound imaging system during the next half hour [ 179 ], with repeated ultrasound/microbubble treatment cycles administered with every scheduled administration of gemcitabine, up to 32 weeks. Expansion of this trial to a larger group of patients now points towards the beneficial extension of life for the patients undergoing this treatment, with 60% surviving 12 months [ 180 ].
Bioeffects of ultrasound in therapeutic applications
Therapeutic applications of ultrasound may span beyond thermal or mechanical disruption of the tissues or drug delivery. Action of ultrasound on the tissues, possibly in combination with microbubbles, may lead to manifestation of a variety of therapeutic bioeffects, spanning from therapeutic angiogenesis [ 181 ] to inhibiting blood flow in the tumors [ 182 – 184 ], to targeting therapeutic stem cells following intravenous administration [ 185 ], bone fracture healing [ 186 ], and, surprisingly, ultrasound action on splenic nerve to mitigate acute kidney injury [ 187 , 188 ]. Non-invasive brain stimulation by ultrasound is also quite intriguing [ 189 ].
All these techniques are based on the ability of ultrasound (as a pressure wave) to provide guided energy deposition in the treatment area; in some instances the ultrasound action is further enhanced by the presence of vibrating microbubbles. Physiological effects demonstrated by ultrasound application are quite diverse and will definitely lead to the development of new therapeutic approaches and modalities.
Ultrasound has become an indispensable tool of modern medicine that helps expand the borders of radiology. Hardware improvements, based on continuous acceleration of data processing rate, lower cost and smaller size of the electronic devices, are combined with smart transducer design, pulse sequences and novel data processing and analysis schemes. Hand-held and laptop ultrasound is already in wide use, soon to replace cart-based systems. Ultrasound contrast agents bring the ability to monitor tissue perfusion, and targeted agents enable molecular ultrasound imaging of the vascular biomarkers of angiogenesis or inflammation. The ability to direct ultrasound to the desired areas of the body opens up direct therapeutic applications of this modality, including targeted drug and gene delivery, and thrombolytic therapy enhancement. Overall, ultrasound is rapidly developing both as an imaging and a therapeutic modality.
Acknowledgments
NIH EB016752, EB001826, EB002349, HL090700, HL111077, CA102880, DK093841.
Open Access is an initiative that aims to make scientific research freely available to all. To date our community has made over 100 million downloads. It’s based on principles of collaboration, unobstructed discovery, and, most importantly, scientific progression. As PhD students, we found it difficult to access the research we needed, so we decided to create a new Open Access publisher that levels the playing field for scientists across the world. How? By making research easy to access, and puts the academic needs of the researchers before the business interests of publishers.
We are a community of more than 103,000 authors and editors from 3,291 institutions spanning 160 countries, including Nobel Prize winners and some of the world’s most-cited researchers. Publishing on IntechOpen allows authors to earn citations and find new collaborators, meaning more people see your work not only from your own field of study, but from other related fields too.
Brief introduction to this section that descibes Open Access especially from an IntechOpen perspective
Want to get in touch? Contact our London head office or media team here
Our team is growing all the time, so we’re always on the lookout for smart people who want to help us reshape the world of scientific publishing.
Home > Books > Medical Imaging
Ultrasound Imaging - Current Topics

Book metrics overview
3,808 Chapter Downloads
Impact of this book and its chapters
Total Chapter Downloads on intechopen.com
Total Chapter Citations
Academic Editor
Gregory University , Nigeria
Published 11 May 2022
Doi 10.5772/intechopen.95178
ISBN 978-1-78985-186-1
Print ISBN 978-1-78984-877-9
eBook (PDF) ISBN 978-1-78985-331-5
Copyright year 2022
Number of pages 154
Ultrasound Imaging - Current Topics presents complex and current topics in ultrasound imaging in a simplified format. It is easy to read and exemplifies the range of experiences of each contributing author. Chapters address such topics as anatomy and dimensional variations, pediatric gastrointestinal emergencies, musculoskeletal and nerve imaging as well as molecular sonography. The book is...
Ultrasound Imaging - Current Topics presents complex and current topics in ultrasound imaging in a simplified format. It is easy to read and exemplifies the range of experiences of each contributing author. Chapters address such topics as anatomy and dimensional variations, pediatric gastrointestinal emergencies, musculoskeletal and nerve imaging as well as molecular sonography. The book is a useful resource for researchers, students, clinicians, and sonographers looking for additional information on ultrasound imaging beyond the basics.
By submitting the form you agree to IntechOpen using your personal information in order to fulfil your library recommendation. In line with our privacy policy we won’t share your details with any third parties and will discard any personal information provided immediately after the recommended institution details are received. For further information on how we protect and process your personal information, please refer to our privacy policy .
Cite this book
There are two ways to cite this book:
Edited Volume and chapters are indexed in
Table of contents.
By Solomon Demissie, Mulatie Atalay and Yonas Derso
By Ercan Ayaz
By Haithem Zaafouri, Meryam Mesbahi, Nizar Khedhiri, Wassim Riahi, Mouna Cherif, Dhafer Haddad and Anis Ben Maamer
By Felix Okechukwu Erondu
By Stefan Cristian Dinescu, Razvan Adrian Ionescu, Horatiu Valeriu Popoviciu, Claudiu Avram and Florentin Ananu Vreju
By María Eugenia Aponte-Rueda and María Isabel de Abreu
By Jong Hwa Lee, Jae Uk Lee and Seung Wan Yoo
By J.M. López Álvarez, O. Pérez Quevedo, S. Alonso-Graña López-Manteola, J. Naya Esteban, J.F. Loro Ferrer and D.L. Lorenzo Villegas
By Arthur Fleischer and Sai Chennupati
IMPACT OF THIS BOOK AND ITS CHAPTERS
3,808 Total Chapter Downloads
1 Crossref Citations
2 Dimensions Citations
Order a print copy of this book
Available on

Delivered by
£119 (ex. VAT)*
Hardcover | Printed Full Colour
FREE SHIPPING WORLDWIDE
* Residents of European Union countries need to add a Book Value-Added Tax Rate based on their country of residence. Institutions and companies, registered as VAT taxable entities in their own EU member state, will not pay VAT by providing IntechOpen with their VAT registration number. This is made possible by the EU reverse charge method.
As an IntechOpen contributor, you can buy this book for an Exclusive Author price with discounts from 30% to 50% on retail price.
Log in to your Author Panel to purchase a book at the discounted price.
For any assistance during ordering process, contact us at [email protected]
Related books
Medical imaging.
Edited by Felix Okechukwu Erondu
Medical Imaging in Clinical Practice
Medical and biological image analysis.
Edited by Robert Koprowski
Edited by Yongxia Zhou
Ultrasound Elastography
Edited by Monica Lupsor Platon
New Advances in Magnetic Resonance Imaging
Edited by Denis Larrivee
Frontiers in Neuroimaging
Edited by Xianli Lv
Optical Coherence Tomography
Edited by Giuseppe Lo Giudice
Updates in Endoscopy
Edited by Somchai Amornyotin
Elastography
Edited by Dana Stoian
Call for authors
Submit your work to intechopen.

Endocrine Abstracts
- Issues/Conferences
- Our Services
ECE2006 Poster Presentations Thyroid (174 abstracts)
Ultrasound thyroid changes: Moscow population screening results
Ea troshina , nv mazurina , nv galkina & it martirosyan.
Endocrinology Research Center, Moscow, Russia.
Objective of the study: To determine the prevalence of thyroid tissue changes in mild iodine deficiency region.
Materials and methods: The 17-th month-lasting study was carried out in Moscow colleges, factories and offices. 1520 people (1403 female, 117 male) median age 34 years were screened by thyroid ultrasonography with liner probe 7,5 MHz.
Results: Thyroid ultrasound disorders have been demonstrated to be high prevalent in women 37.6% (527/1403) vs men 13.7% (16/117). As a male cohort was not large enough, only female screening results were evaluated. Nonpalpable focuses of thyroid tissue under 1 cm in diameter were found in 12.5% (176 patients). Thyroid nodules larger than 1 cm were observed in 10.3% (144/1403) patients, 88 (6.3%) had solitary thyroid nodules, 56 (4.0%) had multinodular goiters. Diffuse hypoechogenic thyroid tissue was found in 8.7% (122) patients. Diffuse goiter (with normal echogenic tissue) was revealed in 6.1% (85) patients.
Conclusion: In Moscow (mild iodine deficiency region) the overall prevalence of ultrasound thyroid abnormalities were found 37.6%. We revealed that nonpalpable focuses of thyroid gland under 1 cm are the most prevalent pattern (12.5%) in all age groups. Our study confirms the common established trend: the relative decrease of diffuse goiter prevalence in comparison with nodular goiter (with multinodular goiter predominance) in eldery people (>45 years old).
8th European Congress of Endocrinology incorporating the British Endocrine Societies
Browse other volumes
Article tools
My recent searches, my recently viewed abstracts, troshina ea, mazurina nv, martirosyan it.
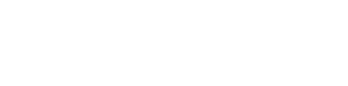
Endocrine Abstracts ISSN 1470-3947 (print) | ISSN 1479-6848 (online) © Bioscientifica 2024 | Privacy policy | Cookie settings
BiosciAbstracts
Bioscientifica Abstracts is the gateway to a series of products that provide a permanent, citable record of abstracts for biomedical and life science conferences.
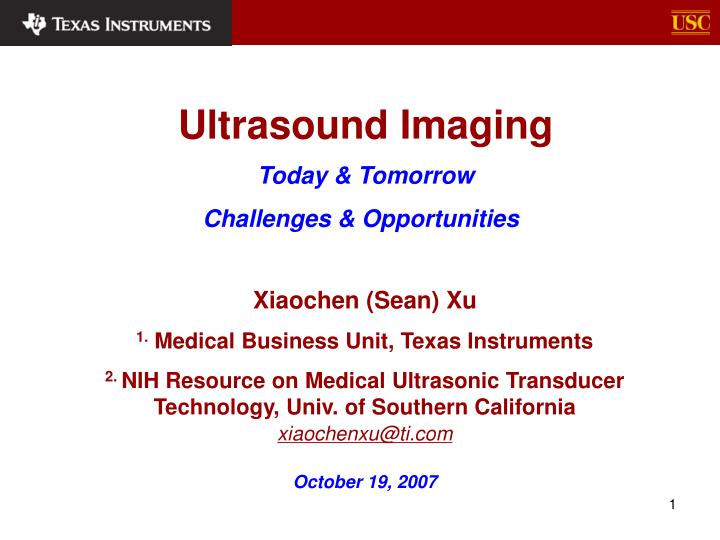
Ultrasound Imaging
Aug 17, 2014
680 likes | 1.42k Views
Ultrasound Imaging. Today & Tomorrow Challenges & Opportunities. Xiaochen (Sean) Xu 1. Medical Business Unit, Texas Instruments 2. NIH Resource on Medical Ultrasonic Transducer Technology, Univ. of Southern California [email protected] October 19, 2007. Background. 15M grant available
Share Presentation
- brightness mode
- nih medical transducer center
- better contrast
- dawei wu dr
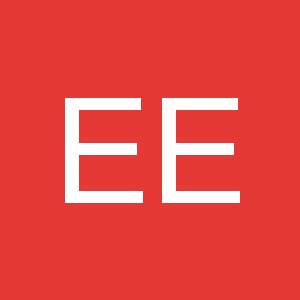
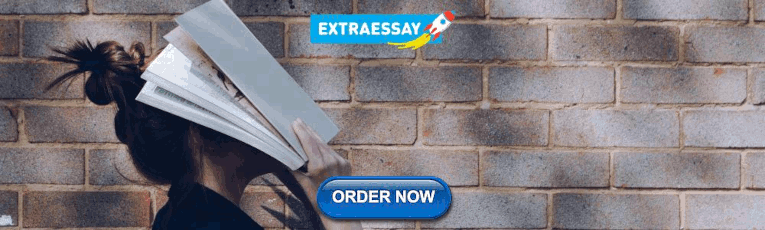
Presentation Transcript
Ultrasound Imaging Today & Tomorrow Challenges & Opportunities Xiaochen (Sean) Xu 1. Medical Business Unit, Texas Instruments 2. NIH Resource on Medical Ultrasonic Transducer Technology, Univ. of Southern California [email protected] October 19, 2007
Background 15M grant available for university medical technology research • TI Medical Business Unit • Personal medical devices • Medical imaging • Wireless healthcare systems • Implantables • NIH Medical Transducer Center • PI: Prof. K. Kirk Shung, Fellow of IEEE(93’), ASA,AIUM • Research Areas: Materials, Transducers, and Systems • Collaborations: 10+ Universities, 5+ Companies. • Personnel: 15+ at USC, 10+ at Penn. State Univ. http://www.ti.com/corp/docs/landing/medtechresearch/index http://bme.usc.edu/UTRC/
Outline Introduction Ultrasound Imaging: Today Ultrasound Imaging: Tomorrow Opportunities & Challenges for SC Summary
Introduction Positron emission tomography • Medical Imaging X-ray, Ultrasound, PET, MRI Magnetic Resonance Imaging Courtesy of google search
Introduction • Ultrasound Imaging • Real-time • Non-invasive • Inexpensive and indispensable • 2~3 billion annual market in the US • New application fields • New technologies
Tx Xducer Target Rx Introduction • Ultrasound • Sound: Mechanical wave, >20KHz, 2-15 MHz • Transducer: • Piezoelectric effects • Mechanical wave Electrical wave • Pulse-echo Imaging mode Signals on Transducer
Active Aperture Oil Direction of electronic scanning Focus 1 Focus 2 Introduction • Transducers Focus 1 Mechanical Sector Scanner Linear Array Phase Array
2r zf Introduction • Transducers • Lateral Resolution • Axial Resolution • Engery • Sensitivity, Vpp, cycle No., Duration, FDA limit
Introduction • Beamformer • Analog Beamformer • Early BF • Low Cost • Digital Beamformer • Flexable • Better performance • Dominant in Market
Introduction • Typical System Courtesy of GE
Ultrasound Imaging: Today • Basic Imaging modes • Brightness Mode (B-mode) • Doppler Mode (D-mode) • Color Doppler Mode (2-D Doppler) • 3D & 4D Ultrasound Courtesy of GE
Ultrasound Imaging: Today • Basic Algorithms • Scan conversion • cFFT based Doppler processing • Autocorrelation for Color Doppler • Image processing • 3-D image Reconstruction
Ultrasound Imaging: Today • Basic Algorithms: Scan conversion Input Data: 30 frame/sec, 2K*256*12bit/frame, ~30M bytes Output Data:30 frame/sec, 512*512/frame(grayscale or color)
sin I CH RF A/D cFFT Q CH cos Time Domain Frequency Domain Q(i) Q(i-1) Φi Φi-1 I(i-1) I(i) Ultrasound Imaging: Today • Basic Algorithms: • cFFT: Directional Doppler Processing • Autocorrelation: Color Doppler Processing
Ultrasound Imaging: Today • Advanced Imaging Modes • Harmonic Imaging • f0 2f0 improved resolution • Better contrast • Contrast Agent • Injection of air-bubble • Improvement of SNR • Enhanced harmonic imaging • Future targeted contrast-agent Courtesy of medscape and Biomed Imaging Interv J 2006; 2(2):e17
Ultrasound Imaging: Today • Advanced Imaging Modes • Coded-excitation • Excite transducer with long pulse • Increase energy i.e. Increase SNR & penetration • Remain same axial resolution Chiao & Hao, IEEE UFFC, vol. 52, no. 2, Feb. 2005
Ultrasound Imaging: Today • Advanced Imaging Modes • Coded-excitation • Barker, Chirp, Golay • More coded algorithms NEEDED Chiao & Hao, IEEE UFFC, vol. 52, no. 2, Feb. 2005
Ultrasound Imaging: Tomorrow • Better Performance • High resolution • Wide application areas • Lower Cost • New transducer technology • New integrated-circuits • Smaller Size • New markets: ambulance, ER, OR • Large volume products
Ultrasound Imaging: Tomorrow • Better Performance • High resolution B-mode Imaging: 75μm at 50 MHz, <15μm Ultrasound Doppler: <0.5 mm/s in capillaries Less Penetration: <1 cm at 50 MHz Areas: dermatology, ophthalmology, small animal
Ultrasound Imaging: Tomorrow • Better Performance • Small Animal Applications • Preferred animal model (mice and rats) • Fast heart rate • Small physical size • Increasing market (Visualsonics, 25M+ Revenue) • Ophthalmology Applications • Retinal vein occlusion • Cornea measurement in LASIK
Ultrasound Imaging: Tomorrow • Better Performance • HF System with 30MHz arrays • First HF array 30MHz • First HF Doppler with array • First HF High-frame System
Ultrasound Imaging: Tomorrow • Better Performance • HF System with 30MHz arrays
Ultrasound Imaging: Tomorrow • Better Performance • HF System with 30MHz arrays 30 Frames/s Rabbit eye images with 30 MHz 64-element Array
Ultrasound Imaging: Tomorrow • Better Performance • HF System with 30MHz arrays Doppler Images 400 Frames/s Mouse heart images with 30 MHz 64-element Array
Ultrasound Imaging: Tomorrow • Better Performance • HF System with Needle Transducer • Retinal vein occlusion (>0.5%) • Evaluating occlusion removal surgery • Velocity change vs. Occlusion • US Doppler with needle transducer Courtesy of www.mrcophth.com
Ultrasound Imaging: Tomorrow • Better Performance • HF System with Needle Transducer
Ultrasound Imaging: Tomorrow • Lower Cost • Expensive Transducers • Expensive Cables (256 1024 shielded wires) • Multi-channel LNA+VCA+ADC+FPGA+DSP
Ultrasound Imaging: Tomorrow • Lower Cost • New Transducer Technology cMUT (capacitive micromachined US transducers) with integrated AFE PZT Mechanical Diced Transducer
Ultrasound Imaging: Tomorrow • Lower Cost cMUT Status GE & Siemens • Reduce Cable Count • 1D 2D i.e. 256256*256 wires in cable • Integrated AFE to transducer • Eliminating impedance mismatch • Eliminating cable insertion loss • Example: • 2D array 2.5 MHz: 39 dB sensitivity improvement US Patent 5744898
Ultrasound Imaging: Tomorrow • Smaller Size • New point-of-care applications • Ambulance, ER, bedside care • Increasing Market 41%growing 2006, 1 billion by 2012 Courtesy of GE and Acuson
Opportunities & Challenges • Growing Portable Ultrasound Market • GE 174M • Sonosite 171M 500M1 Billion in 5 years • Zonare 160M • Compactable and Powerful with Low Price • ASIC Off-shelf ICs • Advanced ICs • More Channel Counts • Semi-conductor Industry • Volume Counts • New Business from cMUTs
Opportunities & Challenges • Wide Operation Voltage • 200 Vpp to 1.8V • Power Dissipation • <100mW/Ch, LNA+VCA+ADC • Low noise & Low power • FPGA+DSP+LCD ~10W • Laptop size PDA size • Heat Issues • Transducer inefficiency: bad to patients and itself • Heat dissipation in smaller systems
Summary “Hand-carried ultrasound systems (HCU) set to transform the ultrasound industry landscape . The HCU market is driven by two technology trends - advanced PC-based architecture and rapid miniaturization. Hand-carried ultrasound systems can reshape the U.S. ultrasound market in two ways and the industry participants will ultimately decide the direction of the market.” —U.S. Ultrasound Markets by Frost & Sullivan Research Service
Acknowledgement Guidance Committee Dr. K. Kirk Shung Dr. Richard M. Leahy Dr. Jesse T. Yen Dr. Jonathan M. Cannata Dr. Ellis Meng
Acknowledgement • NIH Resource Center Dr. Qifa Zhou Dr. Lei Sun Dr. Ruibin Liu Dr. Changhong Hu Mr. Jay Williams Dr. Bin Huang Dr. Rachel Bitton Dr. Jin-Ho Chang Mr. Dawei Wu Dr. Emanuel Gottlieb Dr. Jung-woo Lee Mr. Bruce Lai Dr. Johnson Huang Mr. Peter Lee Mr. Joe Han Mr. Hyung-Ham Kim Mr. Charles Sharp Dr. Jianzhong Zhao Ms. S. Sangkatumvong • Doheny Eye Institute Dr. Mark Humayun Mr. Charles DeBoer Dr. Hossein Ameri • The Institute of Genetic Medicine Dr. Laurence Kedes Ms. Yan Bai
Thank You ! Questions?
- More by User
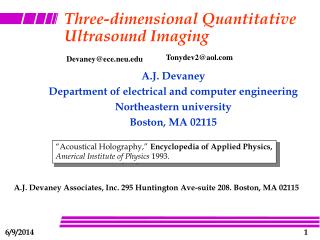
Three-dimensional Quantitative Ultrasound Imaging
Three-dimensional Quantitative Ultrasound Imaging. [email protected]. [email protected]. A.J. Devaney Department of electrical and computer engineering Northeastern university Boston, MA 02115. “Acoustical Holography,” Encyclopedia of Applied Physics, Americal Institute of Physics 1993.
607 views • 15 slides
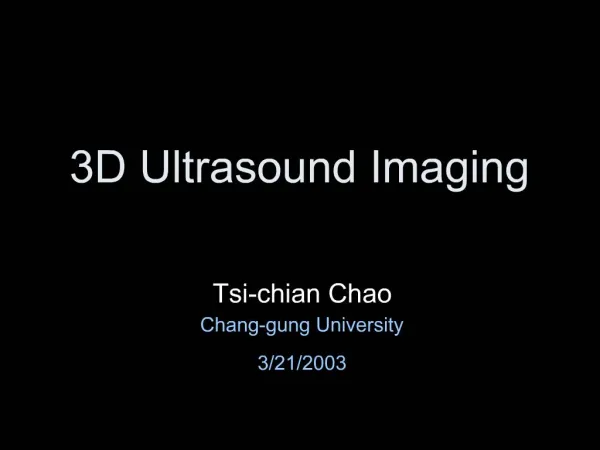
3D Ultrasound Imaging
Historic Review for US. Developed from 1960'HandyReal-timeHarmlessLow costThe most used imaging equipmentNoisyPositioning. The Trends of Medical Imaging. From 2D to 3D.From structural to functional.From post-processing to real time processing.From harmful to safe.From high cost to low cos
1.38k views • 23 slides
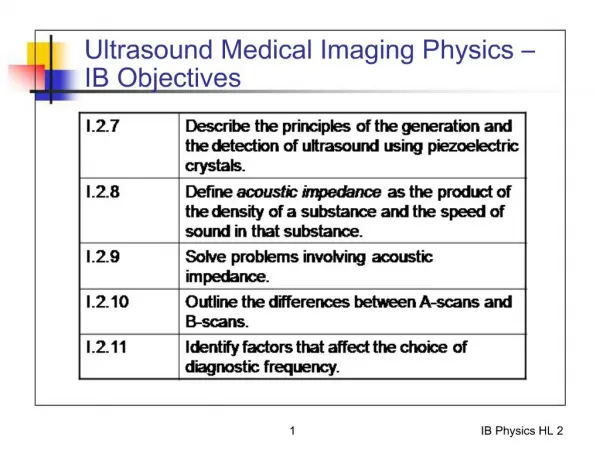
Ultrasound Medical Imaging Physics IB Objectives
775 views • 28 slides
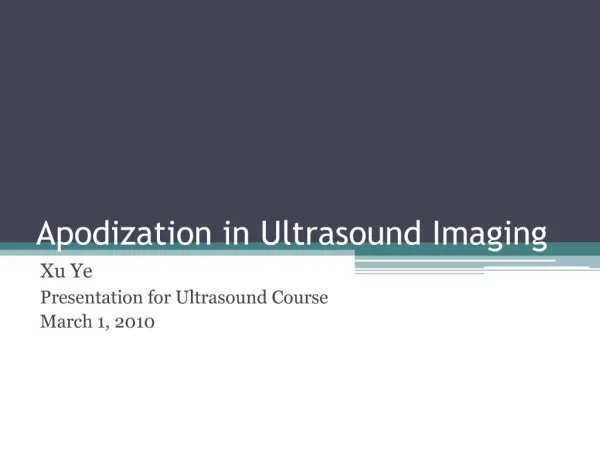
Apodization in Ultrasound Imaging
Contents. ApodizationApodization Functions1D and 2D ApodizationPractical Implementation on Transmit and receiveSingle Element Apodization vs. Array Apodization. Apodization. Narrow the width of the ultrasonic beam produced by the imaging transducer in order to improve the quality of ultrasonic i
1.2k views • 13 slides
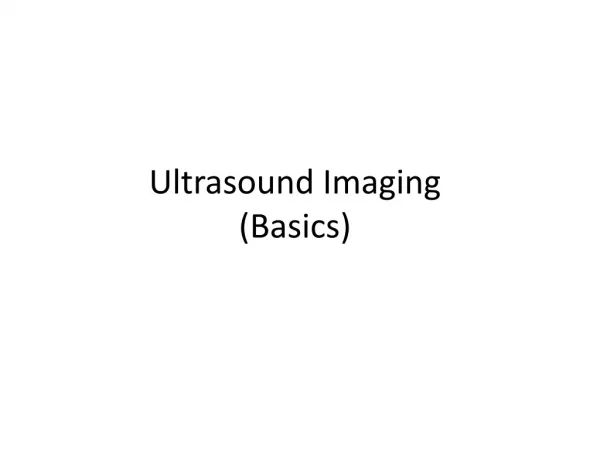
Ultrasound Imaging (Basics)
Ultrasound Imaging (Basics). Why Ultrasound?. Over half a century old technique! Arguably the most widely used imaging technologies in medicine. Portable, free of radiation risk, and relatively inexpensive compared to MRI, CT and PET
1.22k views • 32 slides
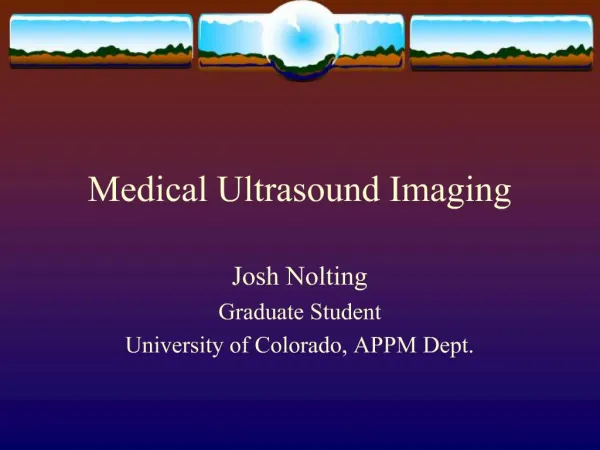
Medical Ultrasound Imaging
Presentation Overview. General ultrasound methodComparison between ultrasound and x-rayHistoryVarious usesPhysics of the methodCurrent stateContinuing technologyConclusion. General method. Place small transducer against the skinEmits high frequency sound wavesDetects bounce back wavesDifferent tissues reflect different wavesReconstruction softwareViewing structure on a screen.
414 views • 18 slides
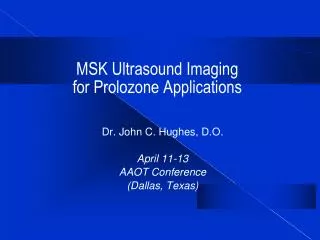
MSK Ultrasound Imaging for Prolozone Applications
MSK Ultrasound Imaging for Prolozone Applications . Dr. John C. Hughes, D.O. April 11-13 AAOT Conference (Dallas, Texas). MSK Ultrasound Visualization and Prolozone Applications. MSK Ultrasound Visualization Tendonitis, Ligament Laxity, Bone Spurs, Trigger Points
917 views • 45 slides
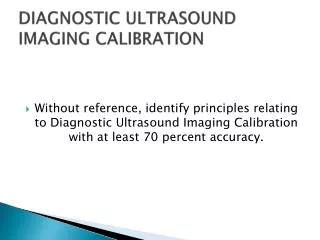
DIAGNOSTIC ULTRASOUND IMAGING CALIBRATION
DIAGNOSTIC ULTRASOUND IMAGING CALIBRATION. Without reference, identify principles relating to Diagnostic Ultrasound Imaging Calibration with at least 70 percent accuracy. . DIAGNOSTIC ULTRASOUND IMAGING CALIBRATION. General Ultrasound Knowledge Other names Real-time scanners
662 views • 16 slides
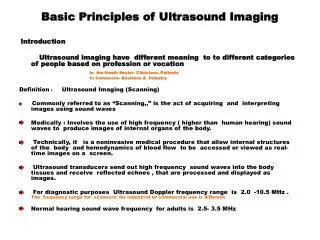
Basic Principles of Ultrasound Imaging
Basic Principles of Ultrasound Imaging. Introduction Ultrasound imaging have different meaning to to different categories of people based on profession or vocation In the Heath Sector- Clinicians, Patients
7.75k views • 17 slides
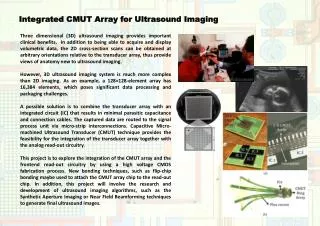
Integrated CMUT Array for Ultrasound Imaging
Integrated CMUT Array for Ultrasound Imaging.
255 views • 1 slides
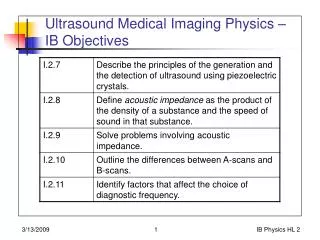
Ultrasound Medical Imaging Physics – IB Objectives
Ultrasound Medical Imaging Physics – IB Objectives. Ultrasound Production and Detection. Based on piezoelectric effect. From http://en.wikipedia.org/wiki/Medical_ultrasonography. Piezoelectric Effect in Crystals. Applied electric field produces mechanical vibration
708 views • 29 slides
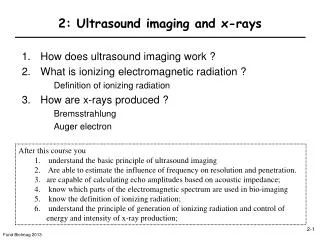
2: Ultrasound imaging and x-rays
2: Ultrasound imaging and x-rays. How does ultrasound imaging work ? What is ionizing electromagnetic radiation ? Definition of ionizing radiation How are x-rays produced ? Bremsstrahlung Auger electron. After this course you understand the basic principle of ultrasound imaging
511 views • 19 slides
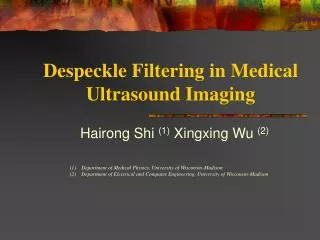
Despeckle Filtering in Medical Ultrasound Imaging
Despeckle Filtering in Medical Ultrasound Imaging. Hairong Shi (1) Xingxing Wu (2). (1) Department of Medical Physics, University of Wisconsin-Madison (2) Department of Electrical and Computer Engineering, University of Wisconsin-Madison. Introduction.
407 views • 29 slides
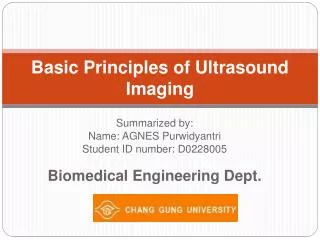
Basic Principles of Ultrasound Imaging. Summarized by: Name: AGNES Purwidyantri Student ID number: D0228005 Biomedical Engineering Dept. Ultrasound. Sound waves above 20 KHz are usually called as ultrasound waves.
1.73k views • 22 slides
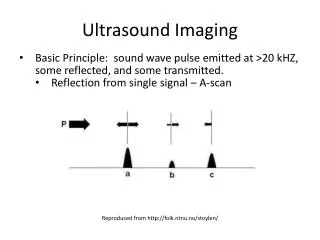
Ultrasound Imaging. Basic Principle: sound wave pulse emitted at >20 kHZ , some reflected, and some transmitted. Reflection from single signal – A-scan. Reproduced from http://folk.ntnu.no/stoylen/. B-Mode Imaging. E nergy displayed as: - amplitude, as in A-mode,
455 views • 4 slides
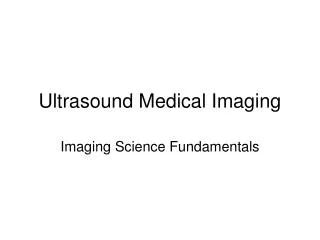
Ultrasound Medical Imaging
Ultrasound Medical Imaging. Imaging Science Fundamentals. Sound waves. Sound waves are mechanical waves. Not in electromagnetic spectrum! Just like light waves, sound waves transmit energy, and can be described in terms of wavelength, period, speed, and amplitude
569 views • 13 slides
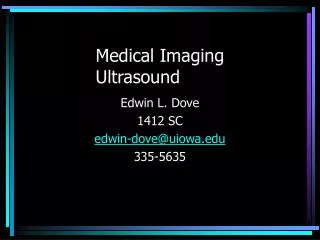
Medical Imaging Ultrasound
Medical Imaging Ultrasound. Edwin L. Dove 1412 SC [email protected] 335-5635. 3D Reconstruction. Why Ultrasound in Cardiology?. Portable, relatively cheap Non-ionizing During the echocardiogram, it is possible for the cardiologist to: Watch the heart’s motion – in 2D real-time
1.23k views • 90 slides
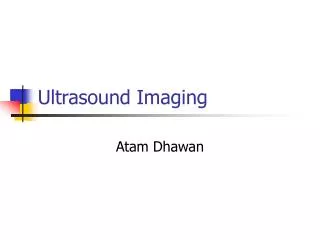
Ultrasound Imaging. Atam Dhawan. Ultrasound. Sound waves above 20 KHz are usually called as ultrasound waves. Sound waves propagate mechanical energy causing periodic vibration of particles in a continuous, elastic medium.
791 views • 21 slides
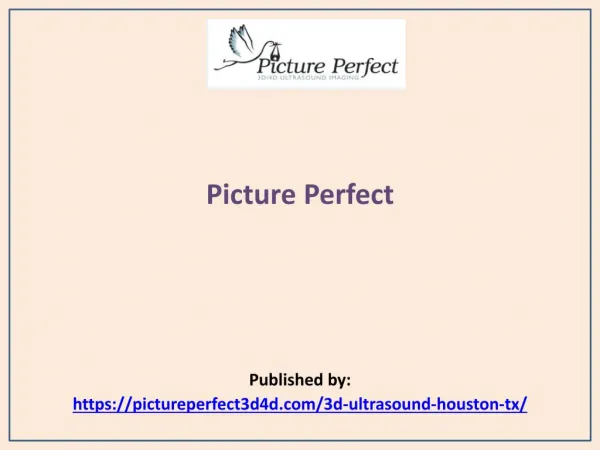
3D/4D Ultrasound Imaging
Picture Perfect is an ultrasound studio run by Evelyn Vasquez and is located at Texas. The 3D/4D sonographic images of the fetus developing inside the mother's womb are created at the studio that presents an unforgettable and rewarding experience. The sonographer Evelyn works in association with the renowned obstetricians in the town and has been serving in the field for more than a decade.
157 views • 5 slides
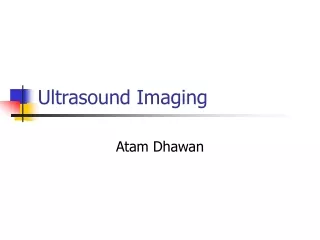
365 views • 21 slides
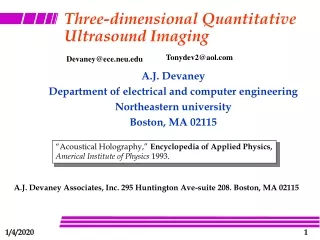
163 views • 15 slides
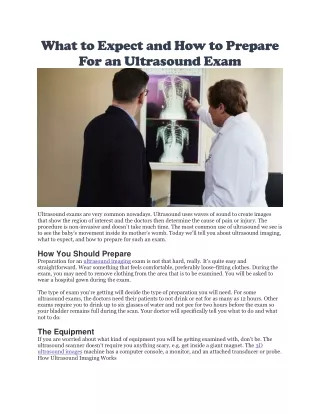
Ultrasound imaging
Preparation for an ultrasound imaging exam is not that hard, really. Itu2019s quite easy and straightforward. Wear something that feels comfortable, preferably loose-fitting clothes. During the exam, you may need to remove clothing from the area that is to be examined. You will be asked to wear a hospital gown during the exam.
59 views • 2 slides
- Unlocking the Potential of Telenursing for...
Radiomics Advances Prognostication in Advance
- Cryocure-VT Trial - Clinical Ventricular...
- Artificial Intelligence in Critical Care:...
- Navigating Connectivity Challenges in Hospita
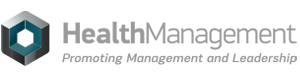
Can GPT-4 Enhance Radiology Workflows by...
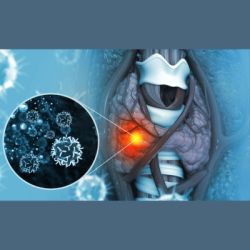
Fatty Acid Metabolism Biomechanical Influence
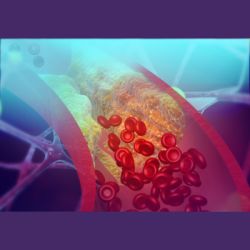
CT-FFR Analysis Lowers Invasive Procedures...
Moscow city department of healthcare chooses agfa healthcare enterprise imaging.
- Tue, 30 Jun 2015
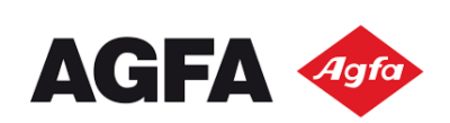
- VNA will consolidate radiology results from multiple sites, while XERO Viewer integrated with the EMR will make them accessible across the Moscow health infrastructure.
- Solution will cover 63 sites with over 110 imaging modalities.
- This is the first multisite imaging IT solution in Russia delivered as a managed service.
Agfa HealthCare has announces that its Enterprise Imaging platform has been chosen by the Moscow City Department of Healthcare as its central imaging repository and teleradiology solution. This is the first multisite imaging IT solution in Russia to be delivered as a managed service. Project implementation began in February 2015, working with local integrator Laval.
Optimizing the radiology workflow across the entire city
The Moscow City Department of Healthcare handles the healthcare needs of both city residents and visitors. The healthcare system includes government and private medical institutions, offering a full range of medical services at the highest level. These days, the health service in Moscow is one of the leading industries of the city, and employs several hundred thousand people. The development of healthcare is constantly supported and funded by the authorities of the capital.
The primary role of the Enterprise Imaging project is to provide optimized radiology workflow in line with approved standards and global best practice s . The solution will cover 63 sites with over 110 imaging modalities, including 59 CT and 39 MRI. State of the art PACS (picture archiving and communication system) and RIS (radiology information system) components will be deployed locally and in the cloud.
Immediate access to consolidated results
Agfa HealthCare's vendor neutral archive (VNA) will consolidate radiology results from multiple sites, while the zero-footprint XERO Viewer integrated with the cloud-based electronic medical record (EMR) will make those results accessible across Moscow's health information exchange (HIE) infrastructure. Integrating the solution with the HIE will enable unique patient identification, allow referring physicians to place orders via the EMR and give authorized users from the Moscow Healthcare community seamless access to results.
An automated radiology workflow has already been provided for the first five connected sites, including the reading center. Local radiology workflow automation will be extended with the support of multi-site teleradiology orchestration. The solution will allow local radiology departments to seamlessly share reading tasks with the dedicated reading center.
The solution, which is based on high availability and disaster recovery best practices, is deployed in two redundant datacenters. Its load-balancing architecture enables to deliver high performance and failover protection.
Proven maturity and high level of expertise
"We are very pleased that the Moscow Department of Health chose Agfa HealthCare for this ground-breaking, multi-site project: the first in Russia in which all deliverables - software components, the centralized hardware platform, the local hardware components, data center and network infrastructure - are all provided by the integrator through a managed services model," says Franz Tiani, Agfa HealthCare's General Manager for Direct Export, Nordic, Central & Eastern Europe. "What's more, the solution can also be extended beyond radiology, to support a consolidation of multimedia results in the VNA. This would allow even more caregivers to collaborate and contribute to a comprehensive patient imaging record in a multidisciplinary environment."
"The proven maturity of the Agfa HealthCare solution made us confident that this was the right choice for a major project of this size and scope," comments Yury Kashkarov, the IT healthcare counselor to the Deputy of Moscow Major responsible for the social development. "Agfa HealthCare has a high level of expertise implementing projects on the enterprise and regional levels, worldwide. This is what we need to ensure the success of this project, which will support us in continuing to enhance the quality of healthcare we provide the people and visitors of Moscow."
Source credit: Agfa HealthCare
«« Imaging Techniques Produce Heart Model
#UKRC2015: Simple Solutions for Voice Recognition Errors »»
Latest Articles
Decision-making in the icu.
- Journal Article
Decision-making in the ICU is a multifaceted process that involves clinical assessment, collaboration among multidisciplinary teams, ethical considerations, evidence-based practice, communication, and continuous adaptation to evolving clinical scenarios. Balancing the complex factors requires
Dealing With Uncertainty in ICU Decision-Making: A Practical Guide
When the stakes are high, and the path ahead is uncertain, the decisions made, especially if a patient continues to worsen, can be sources of self-torment and can haunt us for a long time. Our goal is to suggest ways to steer decision-making for intensivists in the face of uncertainty by prop
The Least Bad Decision: Crisis Standards of Care After the Pandemic
COVID-19 was an emergency that lasted for years and left few regions of the world untouched. The pandemic shone a spotlight on both the strengths and weaknesses of our disaster planning. What worked, what did not, and how can we better plan for future emergencies? The COVID-19 pandemic fo
Related Articles
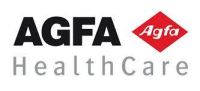
Prime Healthcare Services enters into exclusi
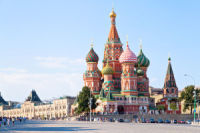
Multisite Moscow Provider Selects Agfa Enterp
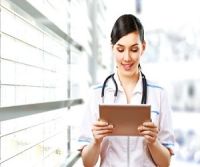
Agfa HeathCare: First Enterprise Imaging...
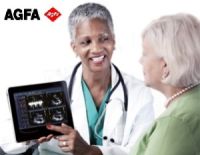
#ESCcongress: Enterprise Imaging by AgfaHealt
Latest news.
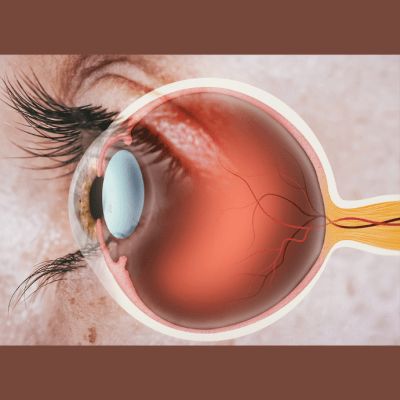
AI-Assisted Ophthalmic Imaging: Enhancing Retinal Cellular Visualisation
In vivo ophthalmic imaging offers insight into individual cell status, but noise reduces contrast, prolonging acquisition time and risking artefacts. Adaptive optics optical coher
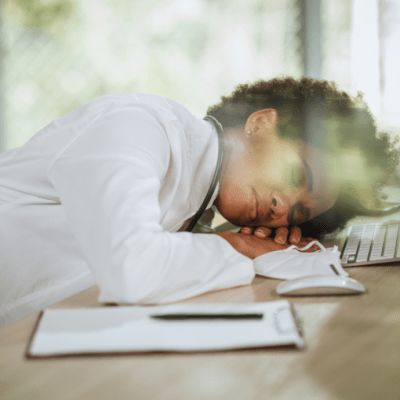
Addressing Burnout and Promoting Well-being in Women Breast Imaging
Addressing Burnout and Promoting Well-being in Women Breast Imaging Burnout, characterised by emotional exhaustion, depersonalisation, and reduced personal efficacy in the...
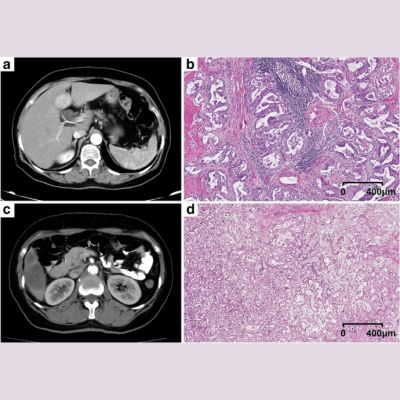
Prognostic Stratification for Liver Cancer Using CT Radiomics
Intrahepatic cholangiocarcinoma (ICC) is the second most common liver cancer after hepatocellular carcinoma, with rising global incidence and mortality rates. Surgical re
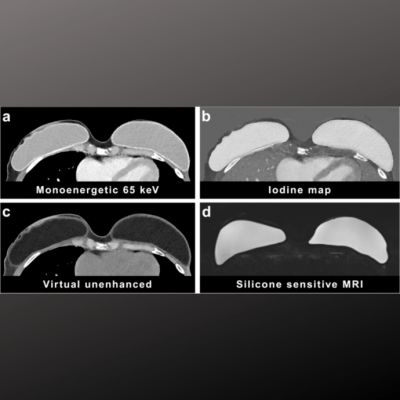
Assessing Silicone Breast Implants Ruptures with PCCT
Breast reconstruction using silicone implants is a common cosmetic procedure for aesthetic enhancement, correcting congenital deformities, or restoring breasts after mas
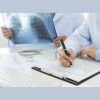
- Movers & Shakers
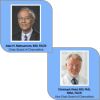
Matsumoto Announced Chair of American College
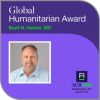
ACR Foundation Presents Global Humanitarian...
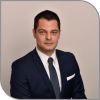
Zisis Sotiriou Joins the Editorial Board...
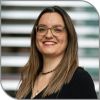
Dr. Christina Malamateniou Assumes Associate...
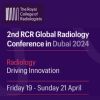
2nd RCR Global Radiology Conference 2024
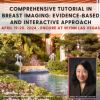
Comprehensive Tutorial in Breast Imaging:...
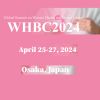
WHBC 2024: Women Health and Breast Cancer
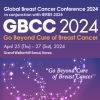
GBCC 2024 - Global Breast Cancer Conference...
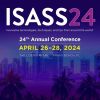
ISASS24 - 24th Annual Conference
- Advertising
- Submit Article
- Author Guide
- Privacy Policy
- Cookie Policy
- Terms and conditions
- Copyright and permissions
- Editorial Board
- White Papers & Case Studies
- IMAGING Highlights
- ICU Highlights
- EXEC Highlights
- IT Highlights
- CARDIO Highlights
- HealthManagement
- ICU Management
- (E)Hospital
- Imaging Management
- Imaging Management French
- Healthcare IT
- Cardiology Management
- IQ - Interventional Quarter
- List your event
- Past Events
- International Association
- National Association
- Universities - Institutions
- Paid Guest Posts
- I-I-I DigiFlash
Communities
- Decision Support
- Women's Health
- Enterprise Imaging
- Artificial Intelligence
- Finance Management
- Cybersecurity
- Sustainability
- Digital Transformation
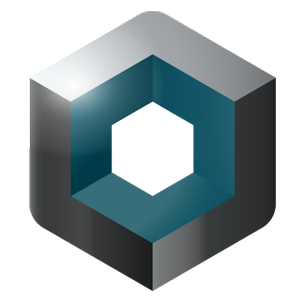
Rue Villain XIV 53-55
B-1050 brussels, belgium, tel: +357 86 870 007, e-mail: [email protected], emea & row office, 166, agias filaxeos, cy-3083, limassol, cyprus, headquarters, kosta ourani, 5, petoussis court, 5th floor, cy-3085 limassol, cyprus.
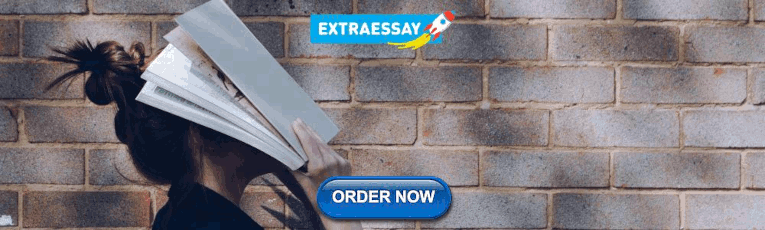
IMAGES
VIDEO
COMMENTS
Ultrasound uses high frequency sound waves to visualize internal structures. It works by transmitting sound waves into the body using a transducer probe, which detects the echoes as they bounce off tissues and organs. The echoes are processed to form images on the ultrasound machine screen in real-time. Common applications include obstetrics ...
Speaker: Dr. Mahan Mathur, MD.Assistant Professor of Radiology and Biomedical Imaging,Yale University School of Medicine
Physical Principles of Ultrasound including Safety. At the end of the lecture you will be able to: Explain how an ultrasound image is generated. Describe the different ultrasound modes used for imaging. Describe the current international safety standards relating to. the thermal index (TI) and the mechanical index (MI)
Presentation Transcript. Basic Principles of Ultrasound Imaging Summarized by: Name: AGNES Purwidyantri Student ID number: D0228005 Biomedical Engineering Dept. Ultrasound • Sound waves above 20 KHz are usually called as ultrasound waves. • Sound waves propagate mechanical energy causing periodic vibration of particles in a continuous ...
Pulse Echo Imaging. Transducer is excited for a short period, generating a narrowband short pulse. Detects backscattered wave (echo) generated by objects. Repeat the above process, with the interval between two input pulses greater than the time for the receiver to receive the echo from the deepest object (2 d_max/c)
One of the most common uses of ultrasound is during pregnancy, to monitor the growth and development of the fetus, but there are many other uses, including imaging the heart, blood vessels, eyes, thyroid, brain, breast, abdominal organs, skin, and muscles. Ultrasound images are displayed in either 2D, 3D, or 4D (which is 3D in motion).
Typical medical ultrasound frequencies are between 2 MHz < ξ < 40 Mhz. Traditionally, medical ultrasound is mainly put to use in diagnostic applications, however, more therapeutic applications are emerging. 11.2. Physics of Sound Waves. This section introduces the basic underlying physics of ultrasound imaging.
Chapter 1: Orientation to Ultrasound Scanning, PowerPoint Presentation; Chapter 2: Ultrasound Principles, PowerPoint Presentation; Chapter 3: Ergonomics: Avoiding Work-Related Injury, PowerPoint Presentation ... Chapter 17: Duplex Ultrasound Imaging of the Lower Extremity Venous System, PowerPoint Presentation; Chapter 18: Duplex Ultrasound ...
UCSF Ultrasound Lecture Series. A monthly one-hour lecture series for sonographers, radiologists, physicians, and other medical professionals involved with the scanning and interpretation of ultrasound images in patient care. This series is approved by the SDMS for CME credit for licensed sonographers (RDMS). A monthly one-hour lecture series ...
The annual world-wide use of ultrasound imaging in practice is already over billions and all pregnant women have been screened with ultrasound in some countries, such as Germany, Norway, Iceland, and Austria for years . In industry, not only portable systems were available since the early 2000s, but also wireless ultrasound imaging transducers ...
Modern imaging equipment has a color-coded arrival time routine, which allows a distinct presentation of the contrast arrival time differential between the tumor and surrounding tissues. ... Molecular ultrasound imaging may be used to assist with image-guided biopsy (e.g., in the breast and prostate cancer setting) and targeted therapeutic ...
Introduction Ultrasound imaging have different meaning to to different categories of people based on profession or vocation In the Heath Sector- Clinicians, Patients Slideshow 2284820 by orsin ... Presentation Overview. General ultrasound methodComparison between ultrasound and x-rayHistoryVarious usesPhysics of the methodCurrent ...
<i>Ultrasound Imaging - Current Topics</i> presents complex and current topics in ultrasound imaging in a simplified format. It is easy to read and exemplifies the range of experiences of each contributing author. Chapters address such topics as anatomy and dimensional variations, pediatric gastrointestinal emergencies, musculoskeletal and nerve imaging as well as molecular sonography. The ...
Ultrasound Imaging. Basic Principle: sound wave pulse emitted at >20 kHZ , some reflected, and some transmitted. Reflection from single signal - A-scan. ... Presentation Overview. General ultrasound methodComparison between ultrasound and x-rayHistoryVarious usesPhysics of the methodCurrent stateContinuing technologyConclusion. General method.
Correct diagnostics of vascular pathologies underlies treatment success for patients with cerebrovascular diseases. Generally, ultrasound is a well-known method for diagnostics of vascular ...
Results: Thyroid ultrasound disorders have been demonstrated to be high prevalent in women - 37.6% (527/1403) vs men - 13.7% (16/117). As a male cohort was not large enough, only female screening results were evaluated. Nonpalpable focuses of thyroid tissue under 1 cm in diameter were found in 12.5% (176 patients).
Monash provided a recognised and well-respected undergraduate degree in medical imaging and a clear pathway into the post graduate medical ultrasound (sonography) degree. We had a great cohort of students in radiography - the relatively small intake size meant that we all got to know each other well, it was a social group where we all found ...
Aug 17, 2014. 680 likes | 1.42k Views. Ultrasound Imaging. Today & Tomorrow Challenges & Opportunities. Xiaochen (Sean) Xu 1. Medical Business Unit, Texas Instruments 2. NIH Resource on Medical Ultrasonic Transducer Technology, Univ. of Southern California [email protected] October 19, 2007. Background. 15M grant available. Download Presentation.
The proceedings of the latest International Congress of Medical Doctors (2000 and 2001), that dealt with new methods of diagnosis, provides evidence of a growing number of NLS-investigations used for the diagnostic purposes - the 2000 summit heard 16 presentations on the subject, while in 2001 there were twice as many.
1. ULTRASOUND IMAGING mlml l Anjan Dangal B.Sc.Medical imaging Technology National Academy of Medical Sciences, Bir Hospital, Kathmandu, Nepal. 2. Application • Ultrasound Imaging is used in following medical working Areas Hospital General Practise Physical Therapy practice Obstetrics centres Veterinary centres. 3.
Department of Healthcare as its central imaging repository and teleradiology solution. This is the first multisite imaging IT solution in Russia to be delivered as a managed service. Project implementation began in February 2015, working with local integr