Thank you for visiting nature.com. You are using a browser version with limited support for CSS. To obtain the best experience, we recommend you use a more up to date browser (or turn off compatibility mode in Internet Explorer). In the meantime, to ensure continued support, we are displaying the site without styles and JavaScript.
- View all journals
- Explore content
- About the journal
- Publish with us
- Sign up for alerts
- Clinical Outlook
- Published: 04 October 2022
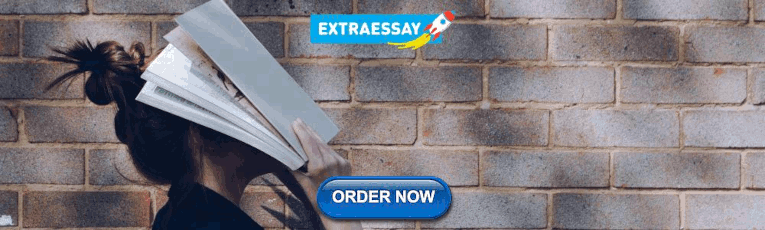
Current and future applications of virtual reality technology for cardiac interventions
- Edris A. F. Mahtab ORCID: orcid.org/0000-0003-2647-5509 1 &
- Anastasia D. Egorova ORCID: orcid.org/0000-0001-9312-2338 2
Nature Reviews Cardiology volume 19 , pages 779–780 ( 2022 ) Cite this article
3317 Accesses
10 Citations
3 Altmetric
Metrics details
- Congenital heart defects
- Interventional cardiology
- Patient education
- Rehabilitation
- Three-dimensional imaging
Virtual reality is a fast-evolving technology that already has several promising applications in medicine. In this Clinical Outlook, we discuss the current evidence and the future challenges for virtual reality applications in cardiac interventions. The incorporation of virtual reality in daily practice will inevitably make clinical care more robust, patient-centred and safe.
Right from its conception in studies of Ibn Sina (also known as Avicenna) and his contemporaries 1 , modern medicine has been and will always be subject to scientific and technological evolution. In the cyber era, the influences of extended reality modalities such as augmented reality and virtual reality (VR) are growing. VR platforms provide the user with the possibility of submerging into an alternative 3D environment and interacting with the surroundings in a real-time manner. A growing number of publications have described the potential applications of VR in medicine. However, randomized controlled trials involving VR in medicine are scarce, and robust evidence for the broad implementation of VR platforms in the clinic is limited. Several methodological and practical challenges need to be addressed in the near future before this highly promising technique can be fully implemented in the field of cardiac interventions (Fig. 1 ).
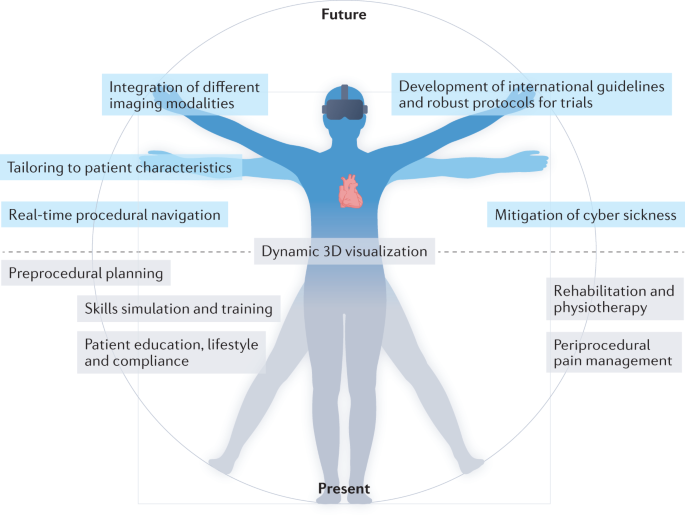
Several 3D virtual reality (VR) modules are in use in the field of cardiac interventions. The applications include skills simulation and training, periprocedural guidance and planning, enhanced 3D imaging visualization, patient education, pain management and rehabilitation. Although the use of VR applications is growing, many technological and methodological challenges remain. These challenges include addressing technical flaws such as the lack of haptic feedback, cyber sickness and the need for integration of multimodality imaging and real-time procedural visualization, as well as the need to tailor the module to the patient. Finally, international guidelines and protocols for the use of VR technology in scientific research and in the clinic are also needed.
Contemporary cardiac care includes increasingly complex percutaneous interventions and technical skills that require iterative practice and many working hours to acquire the necessary dexterity and clinical competency. Interventional cardiology is a continually evolving field associated with numerous technological advances over the past decades. Cardiac interventions are associated with a risk of major complications, which require adequate technical performance and effective team communication under stress to achieve positive outcomes. In this context, lifelong training and sufficient exposure to these cases are imperative, and VR technologies can have an important role in this process.
VR technologies can revolutionize the practice of clinical and technical skills training in the field of cardiac interventions 2 . Many VR-based skills simulators have been described and implemented in practice, including ones for vascular catheter cannulation and angiography, rhythm device and percutaneous valve implantation procedures, procedural anatomy teaching, and patient education and involvement in decision-making. Our group is developing several VR-based simulators for extracorporeal circulation and cardiopulmonary resuscitation after cardiac surgery 3 . These simulations have the potential to boost individual as well as team skills in the setting of complex clinical multidisciplinary situations.
“VR technologies can revolutionize the practice of clinical and technical skills training in the field of cardiac interventions”
VR technology provides digital, online, remotely accessible and blended simulation and live modules. Such a platform can alleviate many of the restrictions imposed by inability to travel (whether owing to travel restrictions, such as during the COVID-19 pandemic, or financial limitations) and provides training support, as well as expert and technical external periprocedural support.
In the preprocedural period, VR technology can provide a diagnostic and planning tool that is both dynamic and interactive. VR simulators facilitate the visualization of complex anatomy and allow the clinician to select the optimal intervention strategy (either percutaneous or surgical), tools and team 4 , 5 . This utility is of particular importance in the field of structural and congenital heart disease, for which interactive 3D visualization of the complex and often unique anatomy is imperative for procedural success 6 , 7 . The first feasibility studies evaluating the integration of CT, MRI and cardiac ultrasonography with VR technology are ongoing. Such technology will allow the incorporation of real-time haemodynamic data in high-spatial-resolution 3D structures. The introduction of these VR modules is expected to change the utilization of diagnostic imaging tools and will enhance our understanding of complex pathoanatomy.
“VR simulators facilitate the visualization of complex anatomy”
The role of VR technology in periprocedural pain management and rehabilitation has also been studied 8 . VR has been shown to reduce patient-perceived pain levels and periprocedural anxiety, as well as contribute to faster post-procedural functional recovery in patients undergoing cardiovascular interventions 9 . Furthermore, VR applications might also be of use as an adjunct strategy in cardiac rehabilitation. However, methodologically robust studies are needed to tailor the technology to the patient group (to take into account factors such as sex, age and technical literacy). Given the growing evidence on the potential of VR modules in both in-hospital and home-based pain management, it is tempting to think that VR technology might indeed become a part of regular treatment plans in the near future 10 . Of note, VR technology incorporates the features of meditation, cognitive behavioural therapy and mindfulness, highlighting how these often underappreciated ‘soft’ concepts of modern medicine can contribute to ‘hard’ clinical outcomes. VR technology can also be incorporated into outpatient clinics to improve patient education and thereby enhance the shared decision-making process and increase patient compliance. Meticulous patient selection and evaluation of cost/benefit ratios require further investigation before VR modules can be successfully implemented in insurance-based health care systems.
The outlook on the use of VR technology for cardiac interventions and patient care is promising. However, several challenges need to be addressed before this technology can be successfully implemented in daily clinical care. Aside from the technical challenges, such as the development of haptic feedback, prevention of cyber sickness, integration of multimodality imaging and implementation of real-time procedural visualization, two drawbacks related to methodology require specific attention. First, the majority of the studies on VR technology have been observational in nature, mostly describing the pioneering work and feasibility of the concept. These studies have low case numbers, single-centre experiences, divergent study protocols and differences in techniques used. The lack of overall methodological standardization makes the comparison of results from these studies challenging and impedes the generation of solid conclusions. Second, no recommendations or a formal consensus is available to guide the incorporation of VR technology in cardiac interventions. The successful implementation of VR in clinical care requires a formalized and, at least to some degree, a standardized approach. To address these challenges, we propose an expert taskforce among international scientific communities (including the AHA, ESC and EACTS) to identify the most important gaps in evidence on the implementation of VR technology in the field of cardiac interventions, as well as to set priorities, standardize research protocols and formulate recommendations and guidelines.
To conclude, the application of VR technology in cardiac interventions is developing apace and is here to stay. These modalities are expected to improve clinical practice and make patient care more robust, patient-centred and safe. However, several technological and methodological challenges need to be addressed before VR platforms can be implemented in the clinic. International cardiovascular communities can have a key role in this process through a dedicated expert taskforce.
UNESCO. Avicenna and the ethics of science and technology today. https://unesdoc.unesco.org/ark:/48223/pf0000134475 (2004).
Pezel, T. et al. Simulation-based training in cardiology: state-of-the-art review from the French Commission of Simulation Teaching (Commission d’enseignement par simulation-COMSI) of the French Society of Cardiology. Arch. Cardiovasc. Dis. 114 , 73–84 (2021).
Article PubMed Google Scholar
Sadeghi, A. H. et al. Virtual reality simulation training for cardiopulmonary resuscitation after cardiac surgery: face and content validity study. JMIR Serious Games 10 , e30456 (2022).
Article PubMed PubMed Central Google Scholar
Jung, C. et al. Virtual and augmented reality in cardiovascular care: state-of-the-art and future perspectives. JACC Cardiovasc. Imaging 15 , 519–532 (2022).
Sadeghi, A. H. et al. Current and future applications of virtual, augmented, and mixed reality in cardiothoracic surgery. Ann. Thorac. Surg. 113 , 681–691 (2022).
Zablah, J. E. & Morgan, G. J. Innovations in congenital interventional cardiology. Pediatr. Clin. North Am. 67 , 973–993 (2020).
Deng, S. et al. A virtual reality system for improved image-based planning of complex cardiac procedures. J. Imaging 7 , 151 (2021).
Birckhead, B. et al. Recommendations for methodology of virtual reality clinical trials in health care by an international working group: iterative study. JMIR Ment. Health 6 , e11973 (2019).
García-Bravo, S. et al. Virtual reality and video games in cardiac rehabilitation programs. A systematic review. Disabil. Rehabil. 43 , 448–457 (2021).
Spiegel, B. et al. Virtual reality for management of pain in hospitalized patients: a randomized comparative effectiveness trial. PLoS One 14 , e0219115 (2019).
Article CAS PubMed PubMed Central Google Scholar
Download references
Acknowledgements
We thank Amir H. Sadeghi for help with preparing this manuscript and Jette J. Peek for help with preparing the figure.
Author information
Authors and affiliations.
Department of Cardiothoracic Surgery, Erasmus Medical Center, Rotterdam, Netherlands
Edris A. F. Mahtab
Center for Congenital Heart Disease Amsterdam Leiden (CAHAL), Department of Cardiology, Leiden University Medical Center, Leiden, Netherlands
Anastasia D. Egorova
You can also search for this author in PubMed Google Scholar
Corresponding author
Correspondence to Edris A. F. Mahtab .
Ethics declarations
Competing interests.
E.A.F.M. is a co-developer of several virtual reality modules in the field of cardiothoracic surgery. A.D.E. declares no competing interests.
Rights and permissions
Reprints and permissions
About this article
Cite this article.
Mahtab, E.A.F., Egorova, A.D. Current and future applications of virtual reality technology for cardiac interventions. Nat Rev Cardiol 19 , 779–780 (2022). https://doi.org/10.1038/s41569-022-00789-4
Download citation
Published : 04 October 2022
Issue Date : December 2022
DOI : https://doi.org/10.1038/s41569-022-00789-4
Share this article
Anyone you share the following link with will be able to read this content:
Sorry, a shareable link is not currently available for this article.
Provided by the Springer Nature SharedIt content-sharing initiative
Quick links
- Explore articles by subject
- Guide to authors
- Editorial policies
Sign up for the Nature Briefing newsletter — what matters in science, free to your inbox daily.

- Perspective
- Open access
- Published: 25 October 2022
Virtual and augmented reality in critical care medicine: the patient’s, clinician’s, and researcher’s perspective
- Raphael Romano Bruno 1 ,
- Georg Wolff 1 ,
- Bernhard Wernly 2 , 3 ,
- Maryna Masyuk 1 ,
- Kerstin Piayda 4 ,
- Susannah Leaver 5 ,
- Ralf Erkens 1 ,
- Daniel Oehler 1 ,
- Shazia Afzal 1 ,
- Houtan Heidari 1 ,
- Malte Kelm 1 , 6 &
- Christian Jung 1
Critical Care volume 26 , Article number: 326 ( 2022 ) Cite this article
12k Accesses
30 Citations
28 Altmetric
Metrics details
Virtual reality (VR) and augmented reality (AR) are aspiring, new technologies with increasing use in critical care medicine. While VR fully immerses the user into a virtual three-dimensional space, AR adds overlaid virtual elements into a real-world environment. VR and AR offer great potential to improve critical care medicine for patients, relatives and health care providers. VR may help to ameliorate anxiety, stress, fear, and pain for the patient. It may assist patients in mobilisation and rehabilitation and can improve communication between all those involved in the patient’s care. AR can be an effective tool to support continuous education of intensive care medicine providers, and may complement traditional learning methods to acquire key practical competences such as central venous line placement, cardiopulmonary resuscitation, extracorporeal membrane oxygenation device management or endotracheal intubation. Currently, technical, human, and ethical challenges remain. The adaptation and integration of VR/AR modalities into useful clinical applications that can be used routinely on the ICU is challenging. Users may experience unwanted side effects (so-called “cybersickness”) during VR/AR sessions, which may limit its applicability. Furthermore, critically ill patients are one of the most vulnerable patient groups and warrant special ethical considerations if new technologies are to be introduced into their daily care. To date, most studies involving AR/VR in critical care medicine provide only a low level of evidence due to their research design. Here we summarise background information, current developments, and key considerations that should be taken into account for future scientific investigations in this field.
Graphical abstract
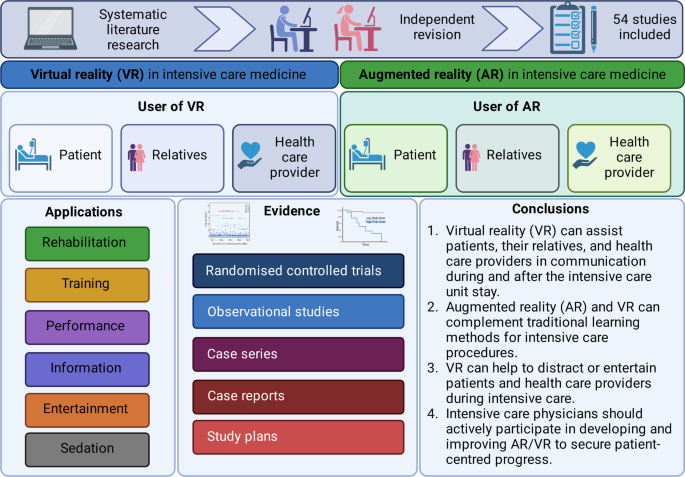
Both, virtual reality (VR) and augmented reality (AR) are technological breakthroughs which facilitate entertainment and communication worldwide [ 1 ]. VR immerses its user completely into a three-dimensional, virtual world, while AR maintains the connection to the “real world” and fuses virtual elements with reality [ 2 ]. VR/AR applications have also gained momentum in critical care medicine. Only recently, Critical Care published E-CHOISIR (Electronic-CHOIce of a System for Intensive care Relaxation), the first cross-over randomised controlled trial that clearly shows the benefits of VR on stress, discomfort, and pain in critically ill patients [ 3 ]. In addition, VR may help providers learn and improve their practical skills in a protected setting [ 4 ], whilst AR offers procedural assistance and continuous surveillance during daily ICU procedures. From a patient’s perspective, VR can alleviate stress, pain [ 5 ], and anxiety [ 6 ] during critical care, and may also promote coordination, mobilisation, physical, and mental rehabilitation. VR has the potential to improve communication between all stakeholder, including relatives, and thus enable coordinated care and understanding. There are numerous potential opportunities for digital VR/AR applications in critical care medicine (see Figs. 1 and 2 , Table 1 ). However, current VR/AR applications have several drawbacks that need refinement. To date there is limited evidence of benefit in this new emerging field of research.
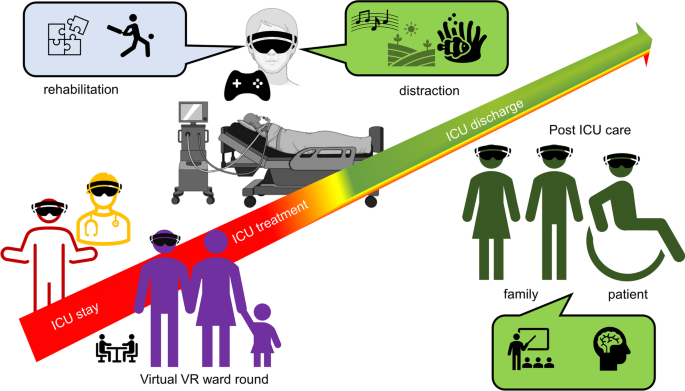
Overview about different users, applications, and the time-course of VR in critical care medicine
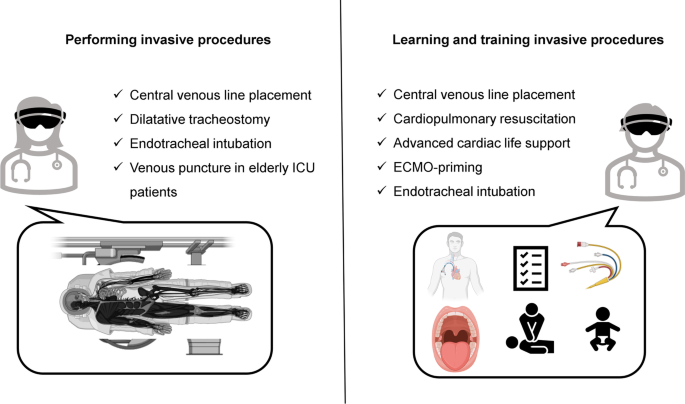
Existing studies where VR/AR applications were used for performing procedures (left panel) and training procedures (right panel)
Virtual reality from the patient’s perspective
Alleviating stress and anxiety.
Patients often experience the ICU as a “hostile” environment due to a number of factors including: excessive noise, loss of self-autonomy and a lack of information [ 5 ]. This is augmented by stress and anxiety, both of which are considered to be significant risk factors for the development of delirium. Delirium occurs in 35% to 80% of non-ventilated/ventilated ICU patients and is associated with an increased length of stay and mortality [ 7 ]. Since pharmacological interventions often have unwanted, and severe side effects, non-pharmacologic options are of utmost importance to treat, and potentially prevent delirium [ 8 ]. ICU stress can be reduced significantly by a calm environment and relaxation techniques. This is an area where VR has been tested. (Fig. 3 ).
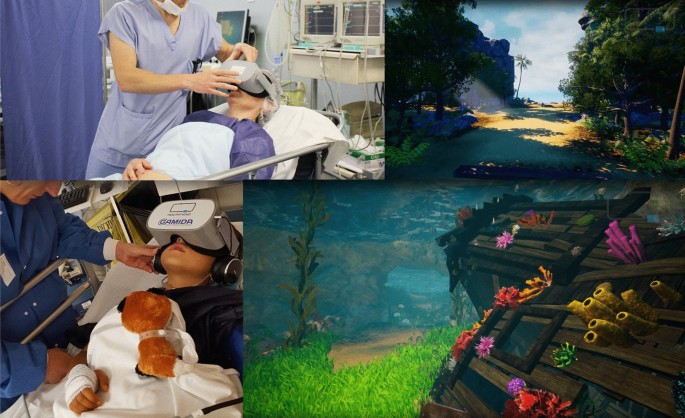
VR with hypnosis used to calm patients during their ICU stay. With permission of Healthy Mind®, France
Rousseaux et al. randomised 100 cardiac surgery patients into four arms (control, hypnosis, VR, and VR combined with hypnosis). Every patient underwent one of the techniques for 20 min the day before and the day after surgery. [ 9 , 10 ]. However, there were no significant differences in the pre-defined outcome measures (anxiety, pain, fatigue, relaxation, physiological parameters, and opioid use) [ 11 ]. Further studies are required to investigate potential beneficial effects, and cost-effectiveness. A relative advantage of VR over hypnosis is that VR does not require additional human resources and does not increase the workload of employed ICU staff. By contrast, the previously mentioned E-CHOISIR (Electronic-CHOIce of a System for Intensive care Relaxation) trial found VR to have a positive effect. Sixty alert, and non-delirious ICU patients were randomised into four relaxation sessions (standard relaxation with television/radio, music therapy, and two virtual reality systems with real motion pictures or synthetic motion pictures). There was a significant decrease in overall discomfort and stress response in the synthetic motion pictures group. Both VR systems led to a reduction in anxiety, but only the synthetic motion pictures group reported lower subjective levels of pain. Three incidents (claustrophobia/dyspnoea/agitation) occurred during the VR sessions, but cybersickness was rare [ 3 ]. Gerber et al. achieved similar results. The investigators used VR with immersive nature scenes in 33 critically ill patients after cardiac surgery. VR acceptance was high, and most patients reported positive effects on stress. These results were supported by a decrease in respiratory rate during VR sessions [ 12 , 13 ]. VR has also been found to have a positive effect on sleep quality: in a randomised-controlled trial of 48 ICU patients, VR use resulted in significantly better sleep quality, although the total sleep time and light sleep time did not differ between the groups [ 14 ].
In the subgroup of paediatric critically ill patients, VR applications have been shown to have a positive effect on stress, anxiety, and delirium. Badke et al. conducted a cross-sectional, single-arm pilot study with 32 paediatric ICU patients who were provided with simple VR headsets and smartphone videos from a widely available multimedia source for distraction [ 15 ]. In this exploratory setting, 82% of parents observed that VR had a calming effect on their child. The same group subsequently recruited 115 critically ill paediatric patients into a comparable study.[ 16 ]. During the VR interaction (median duration: 10 min) the majority of patients and their relatives observed a calming effect. However, children returned to their pre-intervention state once the VR application was stopped.
In conclusion, many studies suggest a positive effect of VR on stress, anxiety, and delirium in critically ill patients. To date, the largest, prospective, randomised-controlled trials in this area have shown neutral [ 11 ] or positive [ 3 ] results.
Virtual reality for pain management
Along with anxiety and stress, pain is one of the most common, and burdensome symptoms in critical care patients. The concept of using VR to distract patients during painful procedures emerged in the late nineteen nineties (Fig. 4 ): There is good evidence for the benefit of VR for the management of chronic [ 17 ] and post-operative pain. Mosso-Vázquez et al. enrolled 67 patients after cardiac surgery. Their VR intervention consisted of different immersive environments [ 18 ]. After VR sessions, 59 patients (88%) reported a decreased level of pain on a Likert Scale. Furthermore, a systematic review and meta-analysis by Ding et al. including eight randomised-controlled trials [ 19 ] found that patients who underwent a VR intervention had lower postoperative pain scores than those receiving standard care. However, there was no significant postoperative pain relief when VR was applied during the pre-operative period. Laghlam et al. evaluated whether VR use in cardiac surgery patients was non-inferior to a combination of nitrous oxide and oxygen. This randomised prospective, non-inferiority, open-label study in 200 patients specifically assessed the degree of pain associated with chest tube removal. VR was inferior to an additionally used inhaled analgetic with regards to the reported level of pain [ 20 ]. Hoffmann et al. tested a VR game in 48 burns victims, age between 6 and 17 years old, while their wounds were cleaned. Compared with the control group, the self-reported pain was significantly reduced [ 21 ]. However, Faber et al. found that the effect of repeated VR interventions might become less effective after three successive days [ 22 ]. According to a study by Hoffman et al. in 11 burn victims, there is a correlation between the “immersive strength” (degree of immersion) of VR and its analgesic effects [ 23 ]. Other research groups additionally focused on the feasibility of VR applications in daily clinical practice. Markus et al. required 59 min for VR setup, instruction, therapy, and cleaning [ 24 ]. In summary, there is convincing evidence for the positive effects of VR on pain management, especially in burn victims and children.
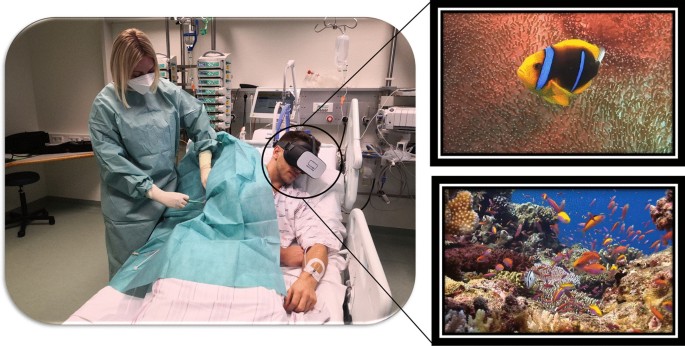
VR for distraction during critical care treatment
Virtual reality for rehabilitation during the intensive care unit stay
“Intensive care unit acquired weakness” during an extended ICU stay is a common phenomenon and has a negative impact on short- and long-term outcomes [ 25 ]. VR applications can support rehabilitation programs on the ICU. Gomes et al. integrated a commercially available gaming platform (Nintendo Wii™) into physical therapy sessions in 60 adult ICU patients, with no mobility restrictions, to enhance their physical activity [ 26 ]. Activity levels were classified as light to moderate on a modified Borg scale. After 100 sessions, 86% of patients stated that they would like to play the videogame in future physical therapy sessions. The same gaming platform (Nintendo Wii™) was evaluated by Abdulsatar et al. in a pilot-trial with 12 critically ill children [ 27 ]. Upper limb activity during Wii™ sessions increased significantly; although grip strength did not change when compared to baseline findings. There were no adverse events attributed to the VR intervention. Although most VR platforms are primarily used in the entertainment industry, specific VR solutions have been designed for health care use. A study conducted by Parke et al. looked to enhance early ICU mobilisation with VR support: 20 adult ICU patients engaged in therapy sessions with the Jintronix virtual therapy system targeting arm, leg, and trunk strength, as well as range of motion, and/or endurance exercises [ 28 ]. The primary objective of this investigation, which was achieved, was safety and feasibility. However, almost all participants reported that the VR activity was enjoyable, improved body strength and range of motion, and would motivate them to continue exercising. ImmersiveRehab® is a commercially available VR environment that uses different tasks to enhance rehabilitation after critical illnesses such as stroke (Fig. 5 ). Additionally, Wang et al. developed a VR application for early mobilisation of critically-ill patients, which has not yet been evaluated in patients or volunteers [ 29 ]. In summary, commercially available VR entertainment applications are safe, feasible and well accepted in critically ill patients and might be beneficial in the physical rehabilitation process on the ICU, although randomised-controlled studies are currently lacking.
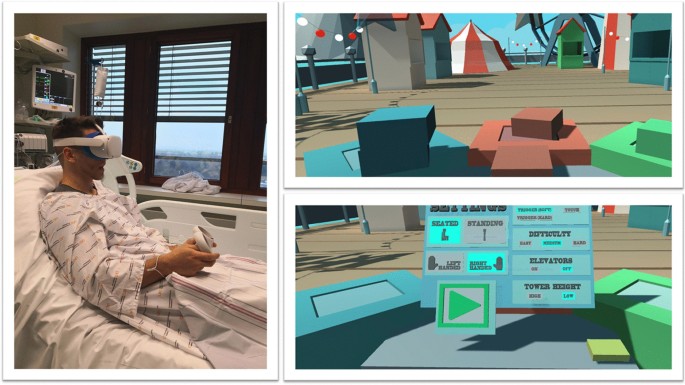
VR with virtual gaming for rehabilitation. With permission from Immersive Rehab Ltd., United Kingdom
Virtual reality for early neurocognitive stimulation
Up to 60% of ICU survivors suffer from significant long-term neurocognitive impairment [ 30 ]. Turon et al. conducted a pilot study on the value of VR-assisted early neurocognitive stimulation in 20 critically ill adult patients undergoing and/or having undergone mechanical ventilation for ≥ 24 h. In brief, the simulation included a virtual avatar that accompanies patients, helps them to orient in time, delivers instructions, motivates them to complete exercises, and encourages them to relax. This VR-assisted neurocognitive intervention was found to be feasible, safe, tolerable, and effectively stimulated cognitive function. However, there was no control group, and no follow-up data were available [ 31 ]. To date, there is no evidence from randomised-controlled trials to support the role of VR in reducing neurocognitive impairment, although promising pilot studies exist.
Virtual reality after intensive care
Following ICU treatment, many patients suffer from Post Intensive Care Syndrome (PICS), which consists of mental health issues, cognitive dysfunction, and problems with mobility [ 32 ]. It was therefore hypothesised that more information on ICU therapy and subsequent medical procedures might be beneficial. Indeed, many ICU patients would like to enhance their knowledge about critical care [ 33 ]. Conventional methods, such as written brochures, are either not well accepted or not utilised [ 33 ]. A randomised-controlled trial by Vlake et al. aimed to determine whether the repetitive application of VR modules explaining ICU treatment improved subjective well-being and quality of life three and six months after ICU treatment. These modules lasted about 14 min and explained different aspects of ICU treatment that were felt to be the most frightening [ 34 , 35 ]. In total, 57 ICU patients were randomised to VR, and 47 patients served as a control group. VR resulted in a reduction of post-traumatic stress disorder and lower depression scores. Mental health was better from two days until one month after initial VR exposure. Interestingly, this effect was still present for post-traumatic stress disorder and depression, but not mental quality of life six months after exposure. Regarding safety, cybersickness scores were low, and no changes in vital signs were observed [ 34 , 35 ]. Recently, the same working group conducted a multicentre randomised-controlled trial including 89 COVID-19 ICU survivors [ 36 ]. The VR strategy consisted of a 14-min informational video with different scenes explaining the ICU environment and treatment. The VR intervention was performed during the COVID-19 post-ICU follow-up clinic appointment, three months after hospital discharge. VR did not reduce the psychological distress or quality of life as compared to the control group. However, VR significantly improved subjective satisfaction scores and the overall rating of ICU aftercare. Most VR patients stated that they would recommend ICU-VR to other ICU survivors. In summary, the use of VR after ICU does not improve clinically relevant endpoints, but has a high acceptance rate among patients.
Virtual reality from the patient relative’s perspective
Situational understanding: virtual intensive care unit rounds.
Admission to a paediatric intensive care unit poses significant stress and uncertainty on relatives—especially the parents. During the COVID-19 pandemic, parents had limited ability to participate in clinical rounds. As a countermeasure, Tallent et al. developed a VR-based virtual visit to the ICU. The VR-visit did not increase the duration of the ward. [ 37 ]. In this study the VR-ICU ward rounds potentially helped to maintain close communication between patients, their relatives, and the health care providers. However, to date, not a single study exists which investigates patient or patient-relative related outcomes in this context.
Virtual reality from the health care provider’s perspective
Virtual reality for education and training.
VR can be used as a tool to train staff how to manage different clinical scenarios and perform clinical skills. [ 2 , 4 ]. VR has some theoretical advantages compared to “real-life training”: complex activities can be repeated as often as desired, no patients or volunteers are required, no company representative is required for instruction, training can be performed at any given time, and no consumable goods are necessary, which might be associated with significant expenditure. For example, when practicing the priming of extracorporeal membrane oxygenation or other cardiac assist devices, considerable material costs can arise per training session.
Multiple studies have been conducted to test the ability of VR to support learning and training of health care providers. In an ICU setting, Chiang et al. evaluated the success of VR-based learning on tracheostomy care in a prospective, controlled, 2:1 randomised pre–post-study. The interventional group ( n = 30) received a VR simulation for 15 min, and the control group regular text-based training. VR increased self-efficacy, including familiarity and confidence, and reduced anxiety about tracheostomy-related knowledge and skills compared to the control group. This effect persisted until three to four weeks after the intervention [ 38 ]. Yu et al. evaluated the impact of a VR simulation program on Korean nursing students’ knowledge, performance self-efficacy, and learner satisfaction in neonatal critical care [ 39 ]. The VR group showed greater improvements in high-risk neonatal infection control performance, self-efficacy and learner satisfaction compared to the control group [ 39 ]. Ralston et al. evaluated a VR environment to test the use of VR in simulating paediatric critically ill clinical scenarios. One scenario simulated an ectopic junctional tachycardia and low cardiac output syndrome; the other simulated an acute respiratory failure in a patient with suspected Covid-19 infection [ 40 ]. Although there was no control group, all six paediatric cardiac critical care physicians successfully navigated the VR environment.
Agasthya et al. evaluated the value of a 19-min immersive tutorial (interventional group) on intubating an infant manikin, in a controlled trial. The primary endpoint (the performance accuracy measured by a checklist) did not differ between groups [ 41 ]. Over 20 years ago, Colt et al. established a VR bronchoscopy simulation for critical care medicine. After VR-training, five novice physicians had comparable skills, in terms of dexterity, speed, and accuracy, to four experienced physicians [ 42 ]. Farra et al. compared the success of VR emergency evacuation training versus web-based clinical updates in a neonatal critical care unit. Both approaches did not statistically differ in their perceived self-efficacy, although the VR group performed statistically better in the live exercise [ 43 ]. Recently, Wolff et al. developed a VR environment consisting of different steps in ECMO-priming (Fig. 6 ) [ 4 ]. In summary, VR or AR might be a complementary, but not a substitution, for training health care providers in basic and advanced life support. In this context, currently available data show heterogeneous results [ 44 ].
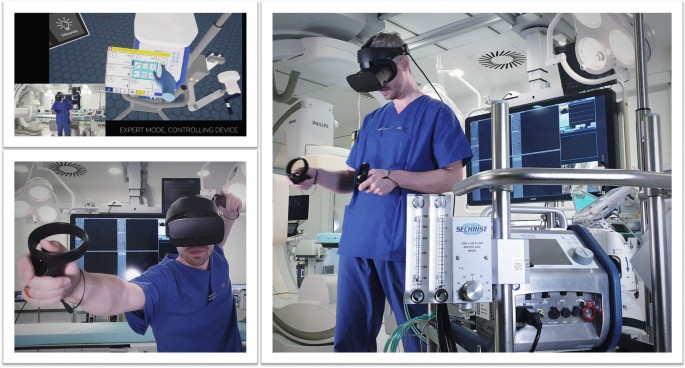
VR for health care providers to train in complex procedures. With permission from Weltenmacher®, Germany
Virtual reality for stress relief
Stress affects ICU health care providers, potentially resulting in burnout and decreased productivity [ 45 ]. Nijland et al. evaluated the impact of VR on the self-perceived stress level of 66 ICU nurses during their breaks. Sixty-two percent of those stated that VR was helpful in reducing stress [ 46 ]. Gerber et al. evaluated the stress relieving effect of VR in 45 healthy subjects: dynamic, virtual, natural, and urban environments were presented inside the head-mounted display and a neutral video on an ICU television screen. The natural environment had the highest positive and restorative impact on the subject’s physiological and psychological state [ 47 ]. Furthermore, ICU caregivers enjoyed pleasant artificial VR environments during their breaks [ 48 ].
Augmented reality for training
AR can assist health care providers in critical care procedures, such as intubation or central line placement. Alismail et al. conducted a controlled trial with 32 ICU trainees. The AR group (15 participants) used head-mounted AR glasses during endotracheal intubation of a training doll. The AR display repeated the essential, practical steps. The interventional group needed more time to intubate and ventilate, but had a higher adherence to evidence-based intubation practice [ 49 ]. Airway management is of pivotal importance in neonatal ICUs [ 50 ]. Dias et al. compared three learning strategies for endotracheal intubation in ICU nurses: direct laryngoscopy, indirect video laryngoscopy and AR-assisted video laryngoscopy with a magnified video of the airway alongside normal vision. AR-assisted video laryngoscopy was not inferior to normal indirect video laryngoscopy and safer than direct laryngoscopy. Huang et al. used a similar AR-based approach for the training of central venous line placement. Although, there was no difference in procedure time, there was a higher adherence to the procedure check list in the AR group ( p = 0.003) [ 51 ]. Heo et al. conducted a prospective, controlled pilot study, randomising nurses with no prior experience in mechanical ventilation to conventional training or AR-assisted training. In the AR-group, the nurses were guided by AR-based instructions and could request assistance using the head-mounted display. AR resulted in a lower need for assistance compared to the manual group and a higher level of confidence after training [ 52 ].
AR can also be used to assess the mental and physical status of patients more accurately and may improve the recognition of deteriorating vital signs. In a trial by Zackoff et al., ICU teams completed two critical care scenarios: first, traditional training using a manikin, then AR-enhanced training using a manikin. AR improved the ability to assess the patient's mental status, respiratory status, and perfusion status, as well as recognition of hypoxemia, shock, apnoea and decompensation, but not the recognition of cardiac arrest.
Augmented reality in performing invasive procedures
Central line placement and endotracheal intubation are standard ICU procedures but can be associated with severe complications. Percutaneous dilatational tracheostomy is a frequently performed intervention on the ICU. In this context, Gan et al. used AR in six patients undergoing the aforementioned procedure with “good success and excellent user feedback” [ 53 ]. The use of an AR-assisted near-infrared electromagnetic radiation device in older ICU patients undergoing venous puncture lowered the incidence of hematomas in venous puncture but did not decrease procedure length or the number of attempts [ 54 ]. Yamada et al. developed an AR interface for smartphones and tablets that can be used by ECMO-perfusionists [ 55 ]. However, to date there are no studies evaluating its effectiveness compared to traditional learning methods. Similarly, Scquizzato et al. proposed an AR based smartphone application for estimating the weight of critically ill paediatric patients, but it has not been evaluated in a clinical setting.[ 56 ]. In conclusion, there is currently no convincing evidence for or against the use of AR-supported invasive procedures in critical care medicine.
AR/VR from a clinician’s perspective
There are a number of promising indications for AR/VR use in critical care medicine, which could be integrated into daily practice. VR could be part of a multimodal strategy, used to reduce analgesic requirements. Likewise, VR may help to support cognitive stimulation and physical activity. However, AR/VR applications are not designed to, and will not be able to, replace personal communication. Patients and their relatives welcome VR-assisted information about ICU procedures [ 57 ]. A similar conclusion applies to VR-based training for health care providers: there are promising approaches to support, but not to replace, traditional learning techniques. To date, there is no convincing evidence for the role of AR-supported practical procedures, such as endotracheal intubation or central venous line placement in critical care medicine outside of clinical trials.
The “vergence accommodation conflict”, cybersickness and possible solutions
VR can cause side effects such as headache, nausea and vomiting—so-called “cybersickness”—which can be related to motion sickness [ 58 ]. Cybersickness is not yet a defined health condition. Motion sickness occurs due to a difference between actual and expected motion. However, this pathophysiological mechanism may not be 100% transferrable to cybersickness. The “vergence accommodation conflict” during VR sessions also plays a role. This phenomenon arises because wearing the VR glasses leads to a disparity between the physical surface of the screen (“accommodation”) and the focal point of the virtual simulated world the user gazes at (“vergence”). This disparity can lead to nausea, headache, and discomfort. At the moment, several possible solutions to the “vergence accommodation conflict” are under evaluation [ 59 ], which potentially challenges the broad application of VR in medical training [ 60 ]. However, cybersickness might be stronger in AR than VR: in one study, 15.3% of participant reported headache and 17 other symptoms, including nausea, after using AR-based training for gross anatomy dissection (HoloAnatomy®) [ 61 ]. By contrast, Bruno et al. found no increased signs of cybersickness during their pilot study, which used VR to distract patients during transcatheter aortic valve implantation [ 6 ]. AR/VR related side-effects seem to vary among different age and gender groups [ 62 ]; an effect which is not yet fully understood and needs further investigation. Thus, the cornerstone of VR-based application might be careful patient selection and prompt assistance should side effects occur.
VR/AR from an ethical perspective
In vulnerable patient groups, such as critically ill patients, there are some ethical concerns regarding the use of VR/AR. For this purpose, Kellermeyer et al. established three core principles [ 63 ]:
If there is a choice, a human-to-human interaction should be preferred (“therapeutic alternativism”) over human-to-machine interaction (no “technological solutionism”).
VR technology should centre around “critical human values,” including dignity and autonomy (“human-oriented value alignment”).
VR systems should be patient centred, not focusing on the need of professional customers (“patient-centered design”).
From our point of view, these principles are of pivotal importance. VR/AR should always enhance the real-world provider—patient-relationship and should not be a tool to replace it. Some researchers proposed the creation of a new medical specialty, the “virtualist”, who undergoes extensive technical and medical training, but also has a deep understanding of the ethical implications of VR/AR technologies [ 64 ]. We believe that critical care physicians and patient representatives should actively participate in the development and continuous improvement of all virtual and digital technologies to ensure they are user-friendly and patient-centred.
VR/AR from a researcher’s perspective
There are a number of difficulties surrounding clinical studies using VR/AR applications. Namely, due to the extensive range of VR/AR glasses (hardware) and software it is extremely difficult to make a direct comparison. In fact, both components are often tested simultaneously in one trial, which may lead to interaction and a lack of clarity in the interpretation of results. [ 65 ]. In future studies, protocols and endpoint definitions should be harmonised as much as possible. The software used differs considerably. Some studies simply use commercially available devices and software (e.g. Nintendo Wii [ 27 ]) while others—such as physicians and researchers—customise the software from existing VR environments to specific patient/educational needs [ 6 ]. Additionally, some manufacturers specifically produce the exact software to create the environment required for the clinical purpose (ImmersiveRehab Ltd or Healthy Mind®). Most studies are "proof-of-principle" approaches focusing on the feasibility and safety of a specific VR/AR application.
Another problem is that VR hardware is rapidly evolving: head-mounted displays are generating ever-higher graphics resolution, easier interactivity, and, thus, greater immersion. Therefore, studies using the latest VR hardware demonstrate greater utility than older devices.
Unfortunately, the degree of immersion and occurrence of cybersickness are rarely measured or reported, although the effect of VR crucially depends on it [ 66 ]. Complex scores have been developed and validated for this purpose. The Simulator Sickness Questionnaire (SSQ), for example, uses 16 questions with four levels of severity to examine "nausea, oculomotor problems and disorientation" [ 67 ].
Currently, there is a lack of prospectively randomised controlled trials in this area of research. In addition, none of the studies were blinded. Theoretically, the intervention group could be compared with a control group, in which “sham VR applications” are used. “Sham VR applications” could consist of using VR glasses with no specific digital content. It is often difficult to distinguish between the relative benefits of immersive VR compared with established non-pharmacological distraction methods such as relaxation techniques or music therapy. At a minimum, investigators should be blinded to reduce bias.
In summary, future studies should consider the following aspects:
Methodical separation of software and hardware.
A detailed statement of the software development and validation process.
Prospective trial design with a randomised-controlled recruitment.
If possible, double blinding, but at least single blinding should be ensured.
Degree of immersion measurement.
Structured recording of "cybersickness" using validated scores.
Descriptive measures of “usual care” in the control group.
Conclusion and future directions
With the ongoing COVID-19 pandemic, innovative VR and AR applications offer new solutions for many aspects of daily critical care medicine. With advancing data transfer speeds; additional applications are emerging, such as remote distance treatment and care. Currently, remote treatments using robotic devices are under development [ 68 ]. This might enable independent, high-quality care in remote locations where expertise is unavailable. We believe that VR and AR will soon become mainstream reality in ICUs all over the globe. To create evidence-based knowledge, particular attention should be paid to consistent research design in further (clinical) trials.
Availability of data and materials
We did not use and individual participant or patient data.
Abbreviations
Three dimensional
- Augmented reality
Cardiopulmonary resuscitation
Computed tomography scan
Extracorporeal membrane oxygenation
Intensive care unit
Transcatheter aortic valve replacement
Post-traumatic stress disorder
- Virtual reality
Bruno RR, Bruining N, Jung C, group V-IS. Virtual reality in intensive care. Intensive Care Med 2022.
Jung C, Wolff G, Wernly B, Bruno RR, Franz M, Schulze PC, Silva JNA, Silva JR, Bhatt DL, Kelm M. Virtual and augmented reality in cardiovascular care: state-of-the-art and future perspectives. JACC Cardiovasc Imaging 2021.
Merliot-Gailhoustet L, Raimbert C, Garnier O, Carr J, De Jong A, Molinari N, Jaber S, Chanques G. Discomfort improvement for critically ill patients using electronic relaxation devices: results of the cross-over randomized controlled trial E-CHOISIR (Electronic-CHOIce of a System for Intensive care Relaxation). Crit Care. 2022;26(1):263.
Article PubMed PubMed Central Google Scholar
Wolff G, Bruno RR, Reiter M, Kantzow B, Kelm M, Jung C. Virtual reality device training for extracorporeal membrane oxygenation. Crit Care. 2020;24(1):390.
Puel F, Minville V, Vardon-Bounes F. What place for virtual reality in the intensive care unit during medical procedures? J Intensive Care. 2021;9(1):30.
Bruno RR, Lin Y, Wolff G, Polzin A, Veulemans V, Klein K, Westenfeld R, Zeus T, Kelm M, Jung C. Virtual reality-assisted conscious sedation during transcatheter aortic valve implantation: a randomised pilot study. EuroIntervention. 2020;16(12):e1014–20.
Article PubMed Google Scholar
Chanques G, Ely EW, Garnier O, Perrigault F, Eloi A, Carr J, Rowan CM, Prades A, de Jong A, Moritz-Gasser S, et al. The 2014 updated version of the confusion assessment method for the intensive care unit compared to the 5th version of the diagnostic and statistical manual of mental disorders and other current methods used by intensivists. Ann Intensive Care. 2018;8(1):33.
Cavallazzi R, Saad M, Marik PE. Delirium in the ICU: an overview. Ann Intensive Care. 2012;2(1):49.
Rousseaux F, Bicego A, Ledoux D, Massion P, Nyssen AS, Faymonville ME, Laureys S, Vanhaudenhuyse A. Hypnosis associated with 3D immersive virtual reality technology in the management of pain: a review of the literature. J Pain Res. 2020;13:1129–38.
Rousseaux F, Faymonville ME, Nyssen AS, Dardenne N, Ledoux D, Massion PB, Vanhaudenhuyse A. Can hypnosis and virtual reality reduce anxiety, pain and fatigue among patients who undergo cardiac surgery: a randomised controlled trial. Trials. 2020;21(1):330.
Rousseaux AF, Dardenne N, Massion PB, Ledoux D, Bicego A, Donneau AF, Faymonville ME, Nyssen AS, Vanhaudenhuyse A. Virtual reality and hypnosis for anxiety and pain management in intensive care units: a prospective randomised trial among cardiac surgery patients. Eur J Anaesthesiol. 2022;39(1):58–66.
Gerber SM, Jeitziner MM, Wyss P, Chesham A, Urwyler P, Müri RM, Jakob SM, Nef T. Visuo-acoustic stimulation that helps you to relax: a virtual reality setup for patients in the intensive care unit. Sci Rep. 2017;7(1):13228.
Gerber SM, Jeitziner MM, Knobel SEJ, Mosimann UP, Müri RM, Jakob SM, Nef T. Perception and performance on a virtual reality cognitive stimulation for use in the intensive care unit: a non-randomized trial in critically ill patients. Front Med (Lausanne). 2019;6:287.
Article Google Scholar
Lee SY, Kang J. Effect of virtual reality meditation on sleep quality of intensive care unit patients: a randomised controlled trial. Intensive Crit Care Nurs. 2020;59: 102849.
Badke CM, Essner BS, O’Connell M, Malakooti MR. An innovative virtual reality experience in the PICU: a pilot study. Pediatr Crit Care Med. 2019;20(6):e283–6.
Badke CM, Krogh-Jespersen S, Flynn RM, Shukla A, Essner BS, Malakooti MR. Virtual reality in the pediatric intensive care unit: patient emotional and physiologic responses. Front Digit Health. 2022;4: 867961.
Jones T, Moore T, Choo J. The impact of virtual reality on chronic pain. PLoS ONE. 2016;11(12): e0167523.
Mosso-Vázquez JL, Gao K, Wiederhold BK, Wiederhold MD. Virtual reality for pain management in cardiac surgery. Cyberpsychol Behav Soc Netw. 2014;17(6):371–8.
Ding L, Hua H, Zhu H, Zhu S, Lu J, Zhao K, Xu Q. Effects of virtual reality on relieving postoperative pain in surgical patients: a systematic review and meta-analysis. Int J Surg. 2020;82:87–94.
Laghlam D, Naudin C, Coroyer L, Aidan V, Malvy J, Rahoual G, Estagnasié P, Squara P. Virtual reality vs. Kalinox® for management of pain in intensive care unit after cardiac surgery: a randomized study. Ann Intensive Care 2021;11(1):74.
Hoffman HG, Rodriguez RA, Gonzalez M, Bernardy M, Peña R, Beck W, Patterson DR, Meyer WJ 3rd. Immersive virtual reality as an adjunctive non-opioid analgesic for pre-dominantly Latin American children with large severe burn wounds during burn wound cleaning in the intensive care unit: a pilot study. Front Hum Neurosci. 2019;13:262.
Faber AW, Patterson DR, Bremer M. Repeated use of immersive virtual reality therapy to control pain during wound dressing changes in pediatric and adult burn patients. J Burn Care Res. 2013;34(5):563–8.
Hoffman HG, Patterson DR, Seibel E, Soltani M, Jewett-Leahy L, Sharar SR. Virtual reality pain control during burn wound debridement in the hydrotank. Clin J Pain. 2008;24(4):299–304.
Markus LA, Willems KE, Maruna CC, Schmitz CL, Pellino TA, Wish JR, Faucher LD, Schurr MJ. Virtual reality: feasibility of implementation in a regional burn center. Burns. 2009;35(7):967–9.
Article CAS PubMed Google Scholar
Dinglas VD, Aronson Friedman L, Colantuoni E, Mendez-Tellez PA, Shanholtz CB, Ciesla ND, Pronovost PJ, Needham DM. Muscle Weakness and 5-year survival in acute respiratory distress syndrome survivors. Crit Care Med. 2017;45(3):446–53.
Gomes TT, Schujmann DS, Fu C. Rehabilitation through virtual reality: physical activity of patients admitted to the intensive care unit. Rev Bras Ter Intensiva. 2019;31(4):456–63.
Abdulsatar F, Walker RG, Timmons BW, Choong K. “Wii-Hab” in critically ill children: a pilot trial. J Pediatr Rehabil Med. 2013;6(4):193–204.
Parke S, Hough CL. A EB: the feasibility and acceptability of virtual therapy environments for early ICU mobilization. Pm r. 2020;12(12):1214–21.
Wang J, Zhang C, Jia Y, Shi C, Choi T, Xiao Q. Development of a virtual reality system for early mobilization of critically ill patients. Stud Health Technol Inform. 2019;264:1805–6.
PubMed Google Scholar
Wolters AE, Slooter AJC, van der Kooi AW, van Dijk D. Cognitive impairment after intensive care unit admission: a systematic review. Intensive Care Med. 2013;39(3):376–86.
Turon M, Fernandez-Gonzalo S, Jodar M, Gomà G, Montanya J, Hernando D, Bailón R, de Haro C, Gomez-Simon V, Lopez-Aguilar J, et al. Feasibility and safety of virtual-reality-based early neurocognitive stimulation in critically ill patients. Ann Intensive Care. 2017;7(1):81.
Voiriot G, Oualha M, Pierre A, Salmon-Gandonnière C, Gaudet A, Jouan Y, Kallel H, Radermacher P, Vodovar D, Sarton B, et al. Chronic critical illness and post-intensive care syndrome: from pathophysiology to clinical challenges. Ann Intensive Care. 2022;12(1):58.
Vlake JH, van Genderen ME, Schut A, Verkade M, Wils EJ, Gommers D, van Bommel J. Patients suffering from psychological impairments following critical illness are in need of information. J Intensive Care. 2020;8:6.
Vlake JH, Van Bommel J, Wils EJ, Korevaar TIM, Hellemons ME, Schut AFC, Labout JAM, Schreuder LLH, Gommers D, Van Genderen ME. Effect of intensive care unit-specific virtual reality (ICU-VR) to improve psychological well-being and quality of life in COVID-19 ICU survivors: a study protocol for a multicentre, randomized controlled trial. Trials. 2021;22(1):328.
Article CAS PubMed PubMed Central Google Scholar
Vlake JH, Van Bommel J, Wils EJ, Korevaar TIM, Bienvenu OJ, Klijn E, Gommers D, van Genderen ME. Virtual reality to improve sequelae of the postintensive care syndrome: a multicenter, randomized controlled feasibility study. Crit Care Explor. 2021;3(9): e0538.
Vlake JH, van Bommel J, Wils EJ, Bienvenu J, Hellemons ME, Korevaar TI, Schut AF, Labout JA, Schreuder LL, van Bavel MP, et al. Intensive care unit-specific virtual reality for critically ill patients with COVID-19: multicenter randomized controlled trial. J Med Internet Res. 2022;24(1): e32368.
Tallent S, Turi JL, Thompson J, Allareddy V, Hueckel R. Extending the radius of family-centered care in the pediatric cardiac intensive care unit through virtual rounding. J Am Assoc Nurse Pract 2021.
Chiang DH, Huang CC, Cheng SC, Cheng JC, Wu CH, Huang SS, Yang YY, Yang LY, Kao SY, Chen CH, et al. Immersive virtual reality (VR) training increases the self-efficacy of in-hospital healthcare providers and patient families regarding tracheostomy-related knowledge and care skills: a prospective pre-post study. Medicine (Baltimore). 2022;101(2): e28570.
Yu M, Yang M, Ku B, Mann JS. Effects of virtual reality simulation program regarding high-risk neonatal infection control on nursing students. Asian Nurs Res (Korean Soc Nurs Sci). 2021;15(3):189–96.
Google Scholar
Ralston BH, Willett RC, Namperumal S, Brown NM, Walsh H, Muñoz RA, Del Castillo S, Chang TP, Yurasek GK. Use of virtual reality for pediatric cardiac critical care simulation. Cureus. 2021;13(6): e15856.
PubMed PubMed Central Google Scholar
Agasthya N, Penfil S, Slamon N. Virtual Reality Simulation for Pediatric Airway Intubation Readiness Education. Cureus. 2020;12(12): e12059.
Colt HG, Crawford SW, Galbraith O 3rd. Virtual reality bronchoscopy simulation: a revolution in procedural training. Chest. 2001;120(4):1333–9.
Farra S, Hodgson E, Miller ET, Timm N, Brady W, Gneuhs M, Ying J, Hausfeld J, Cosgrove E, Simon A, et al. Effects of virtual reality simulation on worker emergency evacuation of neonates. Disaster Med Public Health Prep. 2019;13(2):301–8.
Ricci S, Calandrino A, Borgonovo G, Chirico M, Casadio M. Viewpoint: virtual and augmented reality in basic and advanced life support training. JMIR Serious Games. 2022;10(1): e28595.
Dewa CS, Loong D, Bonato S, Thanh NX, Jacobs P. How does burnout affect physician productivity? A systematic literature review. BMC Health Serv Res. 2014;14:325.
Nijland J, Veling W, Lestestuiver BP, Van Driel CMG. Virtual Reality Relaxation For Reducing Perceived Stress Of Intensive Care Nurses During the COVID-19 pandemic. Front Psychol. 2021;12: 706527.
Gerber SM, Jeitziner MM, Sanger SD, Knobel SEJ, Marchal-Crespo L, Muri RM, Schefold JC, Jakob SM, Nef T. Comparing the relaxing effects of different virtual reality environments in the intensive care unit: observational study. JMIR Perioper Med. 2019;2(2): e15579.
Bodet-Contentin L, Letourneur M, Ehrmann S. Virtual reality during work breaks to reduce fatigue of intensive unit caregivers: a crossover, pilot, randomised trial. Aust Crit Care 2022.
Alismail A, Thomas J, Daher NS, Cohen A, Almutairi W, Terry MH, Huang C, Tan LD. Augmented reality glasses improve adherence to evidence-based intubation practice. Adv Med Educ Pract. 2019;10:279–86.
Dias PL, Greenberg RG, Goldberg RN, Fisher K, Tanaka DT. Augmented reality-assisted video laryngoscopy and simulated neonatal intubations: a pilot study. Pediatrics 2021, 147(3).
Huang CY, Thomas JB, Alismail A, Cohen A, Almutairi W, Daher NS, Terry MH, Tan LD. The use of augmented reality glasses in central line simulation: “see one, simulate many, do one competently, and teach everyone.” Adv Med Educ Pract. 2018;9:357–63.
Heo S, Moon S, Kim M, Park M, Cha WC, Son MH. An augmented reality-based guide for mechanical ventilator setup: prospective randomized pilot trial. JMIR Serious Games. 2022;10(3): e38433.
Gan A, Cohen A, Tan L. Augmented reality-assisted percutaneous dilatational tracheostomy in critically ill patients with chronic respiratory disease. J Intensive Care Med. 2019;34(2):153–5.
Fumagalli S, Torricelli G, Massi M, Calvani S, Boni S, Roberts AT, Accarigi E, Manetti S, Marchionni N. Effects of a new device to guide venous puncture in elderly critically ill patients: results of a pilot randomized study. Aging Clin Exp Res. 2017;29(2):335–9.
Yamada Y, Nakamura T, Yamada M, Maruyama J, Maruyama K, Azuma E. Use of augmented reality to assist teaching for future perfusionists in extracorporeal technology. J Extra Corpor Technol. 2019;51(4):244–7.
Scquizzato T, Landoni G, Carenzo L, Forti A, Zangrillo A. A smartphone application with augmented reality for estimating weight in critically ill paediatric patients. Resuscitation. 2020;146:3–4.
Vlake JH, van Bommel J, Wils EJ, Korevaar T, Hellemons ME, Klijn E, Schut AF, Labout JA, Van Bavel MP, van Mol MM, et al. Virtual reality for relatives of ICU patients to improve psychological sequelae: study protocol for a multicentre, randomised controlled trial. BMJ Open. 2021;11(9): e049704.
Mazloumi Gavgani A, Walker FR, Hodgson DM, Nalivaiko E. A comparative study of cybersickness during exposure to virtual reality and "classic" motion sickness: Are they different? J Appl Physiol (1985) 2018.
Kramida G. Resolving the vergence-accommodation conflict in head-mounted displays. IEEE Trans Vis Comput Graph. 2016;22(7):1912–31.
Vovk A, Wild F, Guest W, Kuula T. Simulator sickness in augmented reality training using the Microsoft HoloLens; 2018.
Wish-Baratz S, Gubatina AP, Enterline R, Griswold MA. A new supplement to gross anatomy dissection: HoloAnatomy. Med Educ. 2019;53(5):522–3.
Rynio P, Witowski J, Kaminski J, Serafin J, Kazimierczak A, Gutowski P. Holographically-guided endovascular aneurysm repair. J Endovasc Ther. 2019;26(4):544–7.
Kellmeyer P, Biller-Andorno N, Meynen G. Ethical tensions of virtual reality treatment in vulnerable patients. Nat Med. 2019;25(8):1185–8.
Oran DP, Topol EJ. The rise of the virtualist. Lancet. 2019;394(10192):17.
Yeung AWK, Tosevska A, Klager E, Eibensteiner F, Laxar D, Stoyanov J, Glisic M, Zeiner S, Kulnik ST, Crutzen R, et al. Virtual and augmented reality applications in medicine: analysis of the scientific literature. J Med Internet Res. 2021;23(2): e25499.
Chow H, Hon J, Chua W, Chuan A. Effect of virtual reality therapy in reducing pain and anxiety for cancer-related medical procedures: a systematic narrative review. J Pain Symptom Manag. 2021;61(2):384–94.
Kennedy RS, Berbaum KS, Lilienthal MG. Disorientation and postural ataxia following flight simulation. Aviat Space Environ Med. 1997;68(1):13–7.
CAS PubMed Google Scholar
Madder RD, VanOosterhout S, Mulder A, Bush J, Martin S, Rash A, Tan JM 2nd, Parker J, Li Y, Kottenstette N, et al. Feasibility of robotic telestenting over long geographic distances: a pre-clinical ex vivo and in vivo study. EuroIntervention. 2019;15(6):e510–2.
Download references
Acknowledgements
We thank Filiz Demirtas, Jonas Diepers, Torge Zense and Lisa Jäger for their assistance.
Open Access funding enabled and organized by Projekt DEAL. This work was supported by the Forschungskommission of the Medical Faculty of the Heinrich-Heine-University Düsseldorf, No. 2018-32 to GW and No. 2020-21 to RRB for a Clinician Scientist Track. No specific funding was received for this work. Furthermore, institutional support has been received by the German Research Council (SFB 1116, B06) as well as the State of North Rhine Westphalia (Giga for Health: 5GMedizincampus. NRW, Project number 005-2008-0055 and PROFILNRW-2020-107-A, TP4).
Author information
Authors and affiliations.
Division of Cardiology, Pulmonology, and Vascular Medicine, Medical Faculty, University Hospital Düsseldorf, Heinrich-Heine-University Düsseldorf, 40225, Düsseldorf, Germany
Raphael Romano Bruno, Georg Wolff, Maryna Masyuk, Ralf Erkens, Daniel Oehler, Shazia Afzal, Houtan Heidari, Malte Kelm & Christian Jung
Department of Internal Medicine, General Hospital Oberndorf, Teaching Hospital of the Paracelsus Medical University Salzburg, Paracelsusstraße 37, 5110, Oberndorf, Salzburg, Austria
Bernhard Wernly
Center for Public Health and Healthcare Research, Paracelsus Medical University Salzburg, 5020, Salzburg, Austria
Department of Cardiology and Angiology, Universitätsklinikum Gießen und Marburg, 35391, Giessen, Germany
Kerstin Piayda
General Intensive Care, St George’s University Hospitals NHS Foundation Trust, London, UK
Susannah Leaver
CARID, Cardiovascular Research Institute Duesseldorf, 40225, Düsseldorf, Germany
You can also search for this author in PubMed Google Scholar
Contributions
RRB, GW and CJ analysed the data and wrote the first draft of the manuscript. KP and SL significantly improved the paper. BW, MM, RE, DO, SA, HH and MK gave guidance, and improved the paper. All authors read and approved the final manuscript.
Corresponding author
Correspondence to Christian Jung .
Ethics declarations
Ethics approval and consent to participate.
Not applicable.
Consent for publication
Competing interests.
The authors declare that they have no competing interests.
Additional information
Publisher's note.
Springer Nature remains neutral with regard to jurisdictional claims in published maps and institutional affiliations.
Rights and permissions
Open Access This article is licensed under a Creative Commons Attribution 4.0 International License, which permits use, sharing, adaptation, distribution and reproduction in any medium or format, as long as you give appropriate credit to the original author(s) and the source, provide a link to the Creative Commons licence, and indicate if changes were made. The images or other third party material in this article are included in the article's Creative Commons licence, unless indicated otherwise in a credit line to the material. If material is not included in the article's Creative Commons licence and your intended use is not permitted by statutory regulation or exceeds the permitted use, you will need to obtain permission directly from the copyright holder. To view a copy of this licence, visit http://creativecommons.org/licenses/by/4.0/ . The Creative Commons Public Domain Dedication waiver ( http://creativecommons.org/publicdomain/zero/1.0/ ) applies to the data made available in this article, unless otherwise stated in a credit line to the data.
Reprints and permissions
About this article
Cite this article.
Bruno, R.R., Wolff, G., Wernly, B. et al. Virtual and augmented reality in critical care medicine: the patient’s, clinician’s, and researcher’s perspective. Crit Care 26 , 326 (2022). https://doi.org/10.1186/s13054-022-04202-x
Download citation
Received : 03 September 2022
Accepted : 12 October 2022
Published : 25 October 2022
DOI : https://doi.org/10.1186/s13054-022-04202-x
Share this article
Anyone you share the following link with will be able to read this content:
Sorry, a shareable link is not currently available for this article.
Provided by the Springer Nature SharedIt content-sharing initiative
- Critical care medicine
Critical Care
ISSN: 1364-8535
- Submission enquiries: [email protected]
- Open access
- Published: 11 September 2023
Virtual and augmented reality in intensive care medicine: a systematic review
- Dominika Kanschik 1 ,
- Raphael Romano Bruno 1 ,
- Georg Wolff 1 ,
- Malte Kelm 1 , 2 &
- Christian Jung ORCID: orcid.org/0000-0001-8325-250X 1 , 2
Annals of Intensive Care volume 13 , Article number: 81 ( 2023 ) Cite this article
5354 Accesses
4 Citations
14 Altmetric
Metrics details
Virtual reality (VR) and augmented reality (AR) are rapidly developing technologies that offer a wide range of applications and enable users to experience digitally rendered content in both physical and virtual space. Although the number of studies about the different use of VR and AR increases year by year, a systematic overview of the applications of these innovative technologies in intensive care medicine is lacking. The aim of this systematic review was to provide a detailed summary of how VR and AR are currently being used in various areas of intensive care medicine.
We systematically searched PubMed until 1st March 2023 to identify the currently existing evidence for different applications of VR and AR for both health care providers in the intensive care unit and children or adults, who were in an intensive care unit because of a critical illness.
After screening the literature, a total of 59 studies were included. Of note, a substantial number of publications consists of case reports, study plans or are lacking a control group. Furthermore, study designs are seldom comparable. However, there have been a variety of use cases for VR and AR that researchers have explored. They can help intensive care unit (ICU) personnel train, plan, and perform difficult procedures such as cardiopulmonary resuscitation, vascular punctures, endotracheal intubation or percutaneous dilatational tracheostomy. Patients might benefit from VR during invasive interventions and ICU stay by alleviating stress or pain. Furthermore, it enables contact with relatives and can also assist patients in their rehabilitation programs.
Both, VR and AR, offer multiple possibilities to improve current care, both from the perspective of the healthcare professional and the patient. It can be assumed that VR and AR will develop further and their application in health care will increase.
Graphic Abstract
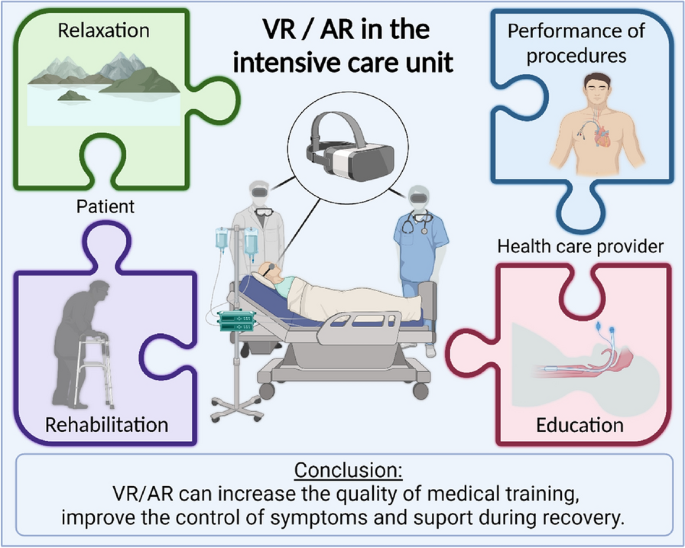
Virtual reality (VR) and augmented reality (AR) are emerging technologies that allow various applications, ranging from immersive entertainment or educational experiences to medical care. VR is defined as the user’s complete immersion into a virtual three-dimensional environment, while AR retains the connection to the real world but supplements it with virtual elements to increase information [ 1 ]. Both VR and AR necessitate special VR/AR glasses for the user. Medical applications are growing and there are already areas that have been intensively researched, such as cardiovascular care [ 2 ] or neurosurgery [ 3 ]. The technologies are also increasingly being used in intensive care medicine and might positively influence this area of medicine from the perspectives of both medical staff and patients [ 4 ]. In a safe environment, VR can help health care providers in acquiring and practice complex intensive care procedures [ 5 ]. Augmented reality can also support the user both before and during procedures by integrating various additional information into reality [ 6 ]. From the patient´s point of view, VR can help to reduce stress during the stay in the intensive care unit through different means, such as distraction from pain, for both adults [ 7 ] and children [ 8 ]. In addition, by combining virtual reality and gaming, it is possible to improve cognitive and motor skills [ 9 ]. Thus, VR and AR could potentially be used at different time points by several users and for different purposes.
The present systematic review presents the current status of the application of VR and AR in critical care medicine. Based on a literature review, we summarized the current state-of-the-art.
Literature search
We systematically searched PubMed databases for publications up until 1st March 2023, applying the following keywords: “VR” and “ICU”, “virtual reality” and “ICU”, "virtual reality" and "critical care", “virtual reality” and “intensive care unit”, "augmented reality" and "ICU, “augmented reality" and "critical care", "augmented reality" and "intensive care", “mixed reality” and “ICU”, "mixed reality" and "critical care", “mixed reality” and “intensive care unit” (Appendix 1) to identify all published studies reporting on the application of virtual or augmented reality in the intensive care unit.
Eligibility and selection criteria
Eligible articles were: randomized controlled trials, nonrandomized trials, observational studies (cases and controls, cohort, and cross-sectional studies), proof-of-concept studies, study protocols, and case reports or series. All studies that met the following criteria were included: (1) type of participants: subjects were either health care providers in the intensive care unit or children or adults, who were in an intensive care unit because of a critical illness. (2) Type of interventions: VR or AR (3) Language: studies published in English or German, both in full text or abstract-only formats.
Data abstraction
Three independent reviewers screened all articles using the above-mentioned inclusion criteria. An independent fourth investigator was involved in the case of discrepancies in the extraction and assessment of the data. The following data were abstracted: author’s name, year of publication, study type, sample size, inclusion criteria, patient characteristics (age, medical background, and treatment), use of AR/VR, frequency of application, and outcomes.
Data synthesis
The key characteristics and results of included studies were summarized and synthesized using tables and complemented by a qualitative summary. This study was conducted and reported following the PRISMA (Preferred Reporting Items for Systematic Reviews and Meta-Analyses) guidelines for reporting systematic reviews [ 10 ].
The initial search strategy identified 786 articles (Fig. 1 ). After the screening on predefined criteria and removal of duplicates, 59 studies were included.
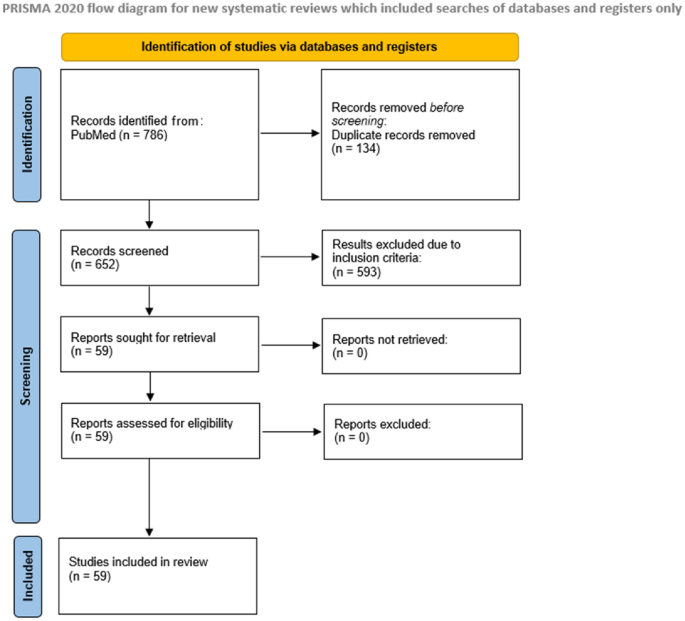
Study flowchart
There were 48 studies about the application of VR: 12 randomized control trials, 2 nonrandomized trials, 26 observational studies, 2 proof-of-concept studies, 4 study protocols and 2 case reports, and 11 studies about the use of AR: 3 randomized control trial, 7 observational studies, and 1 case series.
Table 1 and Table 2 summarize all studies about the use of VR (Table 1 ) and AR (Table 2 ) in intensive care medicine that were included in the search strategy. So all relevant studies are described and commented on in a systematic review. Part 1 focuses on VR and Part 2 on AR. For each perspective, we discuss different applications, both in the adult and pediatric intensive care unit.
VR as a tool for health care providers to improve clinical practice
Adult intensive care medicine.
VR might assist in educating and training healthcare professionals [ 2 ] (Fig. 2 ) as intensive care treatment strategies are often complex and require not only theoretical knowledge but also practical preparation. In a randomized controlled trial of 381 participants, Nas et al. evaluated the value of VR for learning cardiopulmonary resuscitation (CPR). They reported comparable chest compression rates but an inferior compression depth compared with face-to-face training [ 11 ]. The research on VR/AR in this field is generally very heterogeneous [ 12 ]. Wolff et al. developed a VR training environment to improve the traditional training for extracorporeal membrane oxygenation (ECMO) [ 5 ]. Bronchoscopy is another important tool for diagnostic and therapeutic purposes in ICU patients and performing this procedure can be challenging. Colt et al. created a virtual reality bronchoscopy simulation. Through the acquired skills after VR training, five novice physicians were comparable to four experienced physicians regarding dexterity, speed, and accuracy in the model [ 13 ]. In a prospective randomized study with 60 healthcare providers, Chiang et al. evaluated 15-min VR-based learning on tracheostomy care. The use of VR materials increased significantly participants' self-efficacy (increased familiarity, more self-confidence, and less anxiety) and the positive impact persisted until 3 to 4 weeks later [ 14 ].
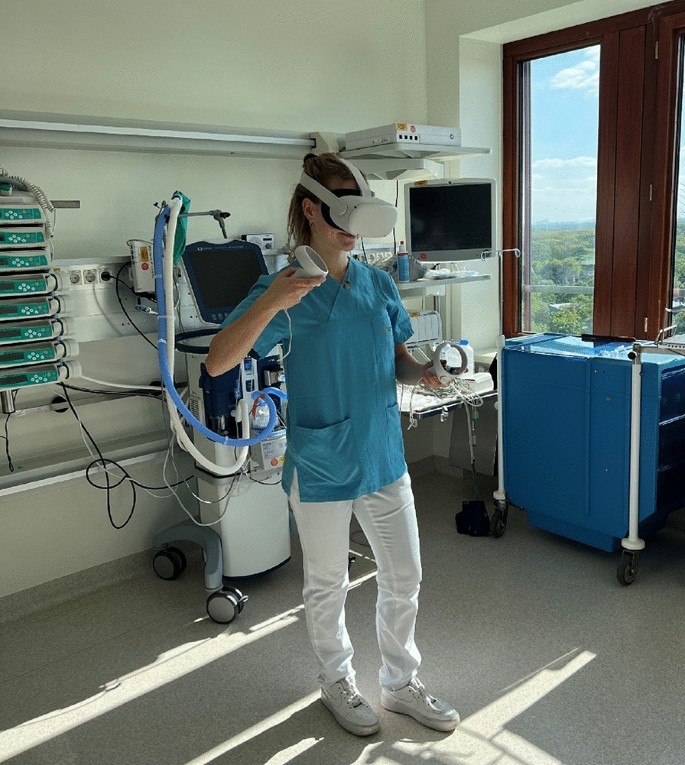
VR application for the training of health care providers
Pediatric intensive care unit
Caring for patients in the neonatal and pediatric intensive care units (PICU) can be particularly challenging [ 15 ], and aspects such as performance, knowledge, self-efficacy, and learner satisfaction are of great importance. In terms of these endpoints, Yu et al. evaluated the effects of a VR simulation program on nursing students. In three scenarios, the interventional group ( n = 25) experienced a 40-min VR simulation and routine practice, and the control group ( n = 25) only did routine practice. The use of VR resulted in a significant increase in high-risk neonatal infection control performance, self-efficacy, and learner satisfaction [ 16 ]. Yang et al. investigated in a non-randomized controlled study the impact of VR neonatal resuscitation program among others on knowledge, problem-solving, or degree of anxiety [ 17 ]. The VR group ( n = 29) participated in a neonatal resuscitation gamification program, while the simulation group ( n = 28) participated in high-fidelity simulations of neonatal resuscitation and online lectures. The control group ( n = 26) had only online lectures on neonatal resuscitation. VR and simulation groups achieved significantly higher levels of neonatal resuscitation knowledge and learning motivation than the control group. Furthermore, VR application was found to be effective in increasing problem-solving ability and self-confidence compared to the others groups. However, anxiety was lowest in the simulation group. Ralston et al. investigated VR-based simulation of two scenarios: ectopic junctional tachycardia and low cardiac output syndrome in the early postoperative period and acute respiratory failure in a patient with suspected coronavirus disease [ 15 ]. All six pediatric cardiac critical care physicians successfully navigated the VR environment and met the critical endpoints such as connect the patient to the pacemaker and correctly overdrive pace or intubate the patient and connect to the ventilator. Farra et al. compared the success of VR training versus web-based clinical updates for emergency evacuation in a newborn ICU. Although there was no significant difference in terms of cognitive assessments and self-efficacy, the VR group performed statistically and clinically better in the live exercise [ 18 ]. Agasthya et al. evaluated a VR tutorial for endotracheal intubation. Participants of the interventional group completed a 19-min immersive guiding and the control group listed the steps from memory. Both groups demonstrated their skills with traditional manikins and were scored on a 24-point checklist. There was no significant difference between the groups [ 19 ].
VR as a tool for healthcare providers to reduce stress
Stress is a common phenomenon in the intensive care unit for both patients and health care providers. In a study with 66 ICU nurses investigated Nijland et al. the effect of VR on perceived stress levels. Sixty-two percent of the ICU nurses, who used VR-Relaxation during their breaktime reported VR to be helpful to reduce stress [ 20 ]. Bodet-Contentin et al. also showed in a study of 88 intensive unit caregivers that the use of VR could improve the efficiency of the breaks [ 21 ].
Patient experiences with VR during and after ICU-stay
Adult intensive care.
From the patients' perspective, intensive care treatment is associated with a number several symptoms such as pain [ 1 ]. If one now modulates attention, environmental conditions, and mood with VR, this can reduce the attention devoted to pain [ 22 ] (Fig. 3 ). Mosso-Vázquez et al. used VR to present different immersive environments such as Cliff or Dream Castle to 67 patients after cardiac surgery [ 23 ]. The results were evaluated with a Likert scale and almost 90% of the patients reported a decreased level of pain experienced post-therapy with VR. Esumi et al. evaluated VR in a patient whose pain after a fasciotomy for acute compartment syndrome could not be adequately controlled and opioid-related side effects, such as respiratory depression, have occurred. The use of VR led to a 25–75% dose reduction in fentanyl administration and the concomitant alleviation of respiratory depression [ 24 ]. In a randomized, prospective study of 200 cardiac surgery patients, Laghlam et al. demonstrated that VR application was equivalent to conventional treatment with oxygen and nitrous oxide in terms of reported pain scores during removal of chest tube [ 25 ]. Markus et al. focused on the technical and procedural feasibility of VR in daily routine and showed that the VR application takes almost an hour for setup, instruction, VR therapy, and cleaning. Especially in smaller centers such programs would be difficult to implement due to lack of staff and resources [ 26 ]. Hoffman et al. demonstrated in their study with 11 burn-injured patients the positive effects of 3-min VR application during wound care on pain relief and a positive correlation between the immersive strength of VR and its pain-relieving effect [ 27 ]. However, Faber et al. showed that the effect would be less after three consecutive days [ 28 ].
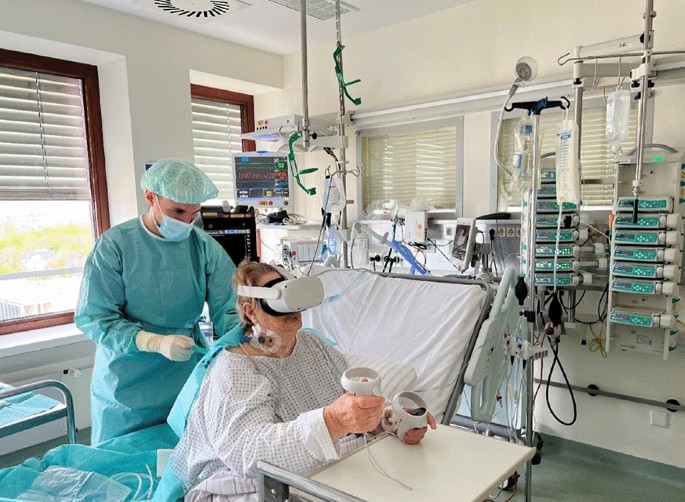
VR application during ICU treatment to distract the patients
Due to discomforts such as aggressive noises, lights, and a lack of information, the intensive care units are often associated with negative feelings such as anxiety or stress for patients [ 7 ]. Merliot‑Gailhoustet et al. investigated in a randomized trial E‑CHOISIR (Electronic‑CHOIce of a System for Intensive care Relaxation) the effects of different electronic relaxation devices on the reduction of overall discomfort, pain, anxiety, dyspnea, thirst, lack of rest feeling and stress in sixty ICU-patients. The patients received four relaxation sessions (standard relaxation with TV or radio, music therapy, and two VR systems with real or synthetic motion pictures). In the group with synthetic motion pictures the overall discomfort, pain, and stress could be significant decrease, while the real motion pictures were associated with a reduction in lack of rest. Both VR-Systems led to a significant decrease in anxiety. Three adverse events might occur: claustrophobia, dyspnea, and agitation. However, in general cybersickness (occurrence of symptoms such as headaches or nausea during VR use) rarely occurred [ 29 ].
Haley et al. evaluated in a pilot study 5-min VR sessions in 10 mechanically ventilated patients. VR therapy proved to be a potential means of managing anxiety in this patient group without the occurrence of predefined safety events or cybersickness [ 30 ]. The quality of sleep could also be positively influenced by the use of VR. In a study with 100 patients, it was shown that due to the VR application, the sleep quality was significantly better but the total sleep time and light sleep time did not differ between the groups [ 31 ].
Hypnosis has been used in the management of acute and chronic pain for a long time [ 32 ]. Rousseaux et al. tested a “virtual reality hypnosis” in patients undergoing cardiac surgery comparing VR to control patients, hypnosis without VR, and VR without hypnosis. All four techniques were used one day before and one day after surgery [ 33 , 34 ]. However, in their randomized-controlled study with 100 patients, there were no significant differences regarding the outcome measures (anxiety, pain, fatigue, relaxation, physiological parameters, and opioid use) between the groups [ 35 ].
To evaluate the usefulness of VR for reducing sensory overload and deprivation in the ICU Jawed et al. put VR goggles on 15 ICU patients for 15 min and exposed them to relaxing beach videos with nature sound effects. Most patients tolerated the headsets well and reported the positive effects of VR therapy on anxiety and stress [ 36 ]. Naef et al. investigated how long visual and auditory stimuli should be provided to intensive care unit patients. In their study, visual stimuli should not exceed 10 – 15 min, while auditory stimuli should not exceed one hour to prevent negative side effects [ 37 ].
Suvajdzic et al. used a combined approach to prevent delirium in ICU patients—The DREAMS system (Digital Rehabilitation Environment-Altering Medical System) [ 38 ], which combined an immersive digital reality acquisition system with a measurement system. The VR environment consisted of a commercially available VR headset. The measurement was sophisticated: it includes physiologic sensors 3-axis wearable accelerometers, a video camera, and environmental sensors for light and noise exposures for measurement of movement, physiologic and emotional responses to assess the movement, physiologic and emotional responses. In addition, an electroencephalogram sensor measures the sleep quality and response to therapy [ 39 ]. The DREAMS system has so far only been used in a small feasibility study with 59 non-intubated ICU patients and was well-received but there was no significant effect on physiologic measures, pain, or sleep [ 40 ].
Family support also plays a big role for the patients in the ICU [ 41 ]. Therefore, He et al. used the fifth generation plus virtual reality (5G + VR) equipment to establish visitation channels for patients and their families during the COVID-19 pandemic. They showed in a cohort study with 141 ICU patients that after 5G + VR visitations, the Hospital Anxiety Depression Scale (HADS) decreased significantly, along with a significant reduction in the proportion of delirium [ 42 ].
ICU patients often experience not only delirium but also other neurocognitive impairments [ 43 ]. In this context, Turon et al. examined in a pilot study the benefits of VR-assisted early neurocognitive stimulation in 20 critically ill adult patients [ 44 ]. The simulation includes a virtual avatar that accompanies patients, helped them orient to time, delivered instructions, motivated them to complete exercises, and encouraged them to relax. This application was found to be feasible, safe, and reliable, and stimulated cognitive functions. Navarra-Ventura et al. evaluated also a VR-based neurocognitive intervention during ICU stay in 34 critically ill patients. A 1-month follow-up that these patients had better working memory scores and showed up to 50% less non-specific anxiety and depression compared to the control group [ 45 ].
Early mobilization of ICU patients improves patient outcomes and reduced hospital stay length [ 46 ]. Gomes et al. used Nintendo Wii™ in 60 adult ICU patients to increase their physical activity [ 47 ]. Activity levels were light to moderate on a modified Borg scale and a majority of patients expressed a desire to play the videogame during their upcoming physical therapy sessions. The study from Parke et al. utilized a similar approach: Xbox Kinect Jintronix software targeting arm, leg, and trunk strength, range of motion, and endurance in 20 adult ICU patients [ 48 ]. Most patients found the activity enjoyable, and easy to understand, as well as motivating to continue participating.
ICU stay constitutes a considerable psychological burden for patients. In several studies, Valke et al. investigated the effects of ICU-specific virtual reality on mental health [ 49 , 50 , 51 , 52 ]. In one of them with 104 patients the group evaluated three and six months after ICU treatment, repetition of 14-min VR modules about ICU treatment improves subjective well-being and quality of life. VR resulted in a reduction of post-traumatic stress disorder, and depression scores, and the effect was still present six months after exposure. Although the mental health was also initially better this effect was no longer observed after six months.
The stay in the pediatric intensive care unit (PICU) can be an emotional and stressful experience for both children and parents [ 53 ]. In a pilot study with 32 critically ill children, Badke et al. investigated the feasibility and satisfaction of virtual reality in the PICU. All participants enjoyed using the technology, and 84% expressed interest in using it for a longer period. The positive effects were also observed among the parents, with 100% reporting satisfaction while watching their children use virtual reality. Moreover, parents reported that their children were calmed by VR [ 54 ]. In another study by this group with 115 critically ill children, the positive influence of VR on engagement and physiologic effects such as heart rate variability was confirmed [ 55 ].
Kucher et al. [ 56 ] and Hoffmann et al. [ 8 ] evaluated VR for better pain management and both were able to show positive effects. Abdulsatar et al. investigated the feasibility and safety of using Nintendo Wii™ in a pilot-trials with 12 critically ill children [ 57 ]. The application improved upper limb activity but grip strength did not change significantly from baseline. Lai et al. used VR on two adolescents suffering from Covid-19. The patients could choose from various active games such as boxing and non-active games such as racing. The authors conclude that VR gaming improved participants’ affect and alertness, motivating them to engage more in early mobilization therapy [ 58 ].
The hospital-induced separation between the child and the family is difficult for both sides. Therefore, Tallent et al. also established a VR-based virtual visit and the staff surveys showed that the application did not lead to an increased duration of the visit. Endpoints on parental perception are not reported, but VR appeared to be very well accepted by the treatment team in this study [ 59 ].
AR as a tool to assist ICU procedures
Adult intensive care unit.
AR can also help health care providers in the implementation of procedures in the ICU. Huang et al. evaluated the AR application during central venous line placement. The AR intervention consisted of a 5- to 10-min hands-on instructional course to allow familiarity with the AR equipment and—during central line placement in a manikin—a video that repeated essential steps. There was no difference between the groups regarding the meantime for placement or procedure time, but a significantly higher adherence level to the checklist between the two groups favoring the AR group was observed [ 60 ]. Fumagalli et al. evaluated the value of AR for venous puncture in 103 ICU patients. The use of AR reduced the incidence of hematomas and anxiety levels but did not reduce the duration of the procedure or the number of attempts [ 61 ]. Morillas Perez et al. also confirmed the positive influence of AR on vascular puncture in a study with 6 operators, who performed a total of 76 punctures. AR application resulted in higher accuracy and better quality of the images and eliminated variability between operators and sonographers. Furthermore, it provided more comfort as the hands are free and the view remains focused on the work area [ 62 ]. In a controlled trial with 32 ICU trainees, Alismail et al. investigated the use of AR during the endotracheal intubation of a manikin. The use of AR, where the essential steps were repeated, resulted in a longer need of time to intubate and ventilate but demonstrated higher compliance with the checklist [ 63 ]. Heo et al. randomized 30 nurses without experience in mechanical ventilation into 2 training groups: with or without AR. Compared to the control group, the AR group requested less assistance and showed higher self-confidence [ 64 ]. Gan et al. evaluated in 6 cases the AR for percutaneous dilatational tracheostomy and again it was confirmed that this new technology allowed the procedure to be carried out successfully [ 65 ]. A pilot study by Zackoff et al. evaluated AR in two critical situations. AR not only improved the ability to assess many factors such as the mental or respiratory status of the patient, but also had a positive impact on the recognition of critical situations such as shock, apnea, and hypoxemia. However, the detection of cardiac arrest was not significantly better [ 66 ]. To improve the training of future perfusionists in the field of extracorporeal circulation (ECC) Yamada et al. developed an AR program for smartphones or tablets [ 67 ]. The AR training might be beneficial for future perfusionists, but currently there has not yet been a clinical study examining the use of the app.
Pediatric intensive
Dias et al. also evaluated AR to improve performing endotracheal intubation. Forty-five participants were randomly divided into three groups and used for intubation on a manikin either direct laryngoscopy or indirect video laryngoscopy or AR-assisted video laryngoscopy. AR-assisted video laryngoscopy was comparable to indirect video laryngoscopy but resulted in increased safety compared with direct laryngoscopy [ 68 ]. The dosage of the drugs used during critical situations in the ICU is often based on weight. Therefore, Scquizzato et al. developed a smartphone app that estimates child weight using the smartphone camera and augmented reality (AR). So far, it has not been evaluated in clinical trials [ 69 ].
Limitations
Although the number of studies about the use of VR or AR significantly increases year by year, attempts at systematic synthesis of evidence such as the present study are limited by scarcely comparable methods, devices, and protocols [ 70 ]. A limited number of prospective randomized controlled trials are currently available in this field and the data are generally very heterogeneous. Thus, quantitative synthesis by meta-analysis and the use of methods to assess the risk of bias in the included studies is hardly possible. Several sources of bias could affect the validity and reliability of studies investigating the use of VR and AR in the ICU. The sample size of the studies is often small and not representative of the overall population of ICU patients, what increases the selection bias. Moreover, the inclusion of older adults may be limited by the fact that they are less familiar with new technologies such as VR/AR and may be hesitant or resistant to trying these innovation methods. It is important that VR/AR interfaces can accommodate age-related changes, such as visual impairments, hearing impairments, and decreased dexterity, to facilitate use the technologies. The performance bias can be high because most studies are not blinded and this can influence the behavior of participants. Furthermore, the outcomes are in most studies subjective and dependent on observation. Establishment of objective evaluation criteria is necessary to improve these aspects. However, there are some subjects such as post-traumatic disorders that are inherently complex and multifaceted, making it difficult to develop such criteria that capture all relevant factors. In addition, a common challenge is the diversity of applied VR and AR systems. This can affect not only the complexity of the application but also the tolerance of the users. VR/AR tools can provide varying levels of user comfort, performance, and immersion. This can make it difficult to reproduce the data, which can reduce the reliability and comparability of the research results. Furthermore, only a few studies describe exactly how time-intensive the application of the technologies can be. This, combined with differences in cost and accessibility, may impact the widespread application of VR/AR.
The present systematic review found the same difficulties for intensive and critical care medicine. In upcoming studies, the protocols should be harmonized as far as possible to expand significant clinical knowledge. The development of a core outcome set plays an important role for future systematic research about VR and AR. This would allow better comparability of studies, improve the quality and relevance of results, and facilitate evidence synthesis and meta-analyses. By providing this information, it would be even more possible to generalize the results and to understand the benefits and limitations of VR in the clinical setting.
This review showed that VR and AR offer new possibilities for many aspects of daily intensive care medicine. There are several approaches to supporting traditional clinical training and taking medical education to the next level. They provide a safe environment to practice procedures such as bronchoscopy [ 13 ], without risking harm to patients. In the ICU, the health care providers often have to make quick decisions, and by simulating different scenarios using VR such as low cardiac output syndrome [ 15 ], the critical decision-making skills can be improved. In addition, the technologies can positively influence aspects such as knowledge or self-efficacy [ 14 , 16 ], and the stress level of hospital staff [ 20 ]. However, the studies also have shown that VR/AR applications do not lead to a significant improvement in the performance of invasive procedures such as central line placement [ 60 ]. Furthermore, VR-based CPR training compared with traditional training provided inferior results [ 71 ].
Although there is currently limited data on clinically relevant outcomes, combining traditional training with VR/AR applications may be the way to achieve the best results in daily clinical practice.
Several studies confirmed that VR also might be an effective tool for pain management. VR allows us to generate a virtual environment to distract patients from their pain for example after surgery [ 23 ] or during wound care [ 8 , 27 ]. As a result, VR therapy can reduce the need for pain medication and thus prevent the occurrence of undesirable side effects of traditional therapy [ 24 ]. Furthermore, the use of VR can lower the stress level and reduce anxiety during the stay in the ICU [ 36 , 72 ]. This can have a positive impact on sleep quality [ 31 ], development of delirium [ 42 ], and cognitive impairment [ 45 ]. Six studies have also shown that VR can help during rehabilitation [ 47 , 48 , 57 , 58 , 73 , 74 ]. The application led to an increase in activity and was well tolerated by the patient without the occurrence of adverse events such as falls.
It is necessary to pay attention to the duration of the application of these technologies because overstimulation can negatively affect the outcome of patients [ 37 ]. Furthermore, cybersickness may occur during the application [ 29 ]. Lastly, the implementation of these technologies into clinical practice requires a significant investment of time by ICU staff [ 26 ], which also may reduce readiness to use them.
Overall, while VR is not a substitute for established therapy, it can be a useful tool in combination with other treatments to improve the patient's stay in an intensive care unit.
Augmented reality (AR) and virtual reality (VR) are no longer the domains of the science fiction world. We are on the verge of making virtual and augmented reality mainstream in the field of medicine and critical care has the potential to be at the forefront of this evolution. However, we cannot forget that VR and AR are not intended to distract us from the patient. They are provided to complement and optimize, but not replace the relationship between a health care provider and a patient. Furthermore, these are still in the research and development phase. Our involvement in this process is important to ensure that these technological developments are made in the best interest of our patients. This makes it possible to provide the best care and to improve the quality of the hospital stay in the ICU.
Availability of data and materials
The anonymized data can be requested from the authors if required.
Abbreviations
Three dimensional
Fifth generation
- Augmented reality
Cardiopulmonary resuscitation
Extracorporeal circulation
Extracorporeal membrane oxygenation
Hospital Anxiety Depression Scale
Head-mounted display
Intensive care unit
Interpupillary distance
Post-intensive care syndrome-family
Preferred Reporting Items for Systematic Reviews and Meta-Analyses
Transcatheter aortic valve replacement
- Virtual reality
Bruno RR, Wolff G, Wernly B, Masyuk M, Piayda K, Leaver S, et al. Virtual and augmented reality in critical care medicine: the patient’s, clinician’s, and researcher’s perspective. Crit Care. 2022;26(1):326.
PubMed PubMed Central Google Scholar
Jung C, Wolff G, Wernly B, Bruno RR, Franz M, Schulze PC, et al. Virtual and augmented reality in cardiovascular care: state-of-the-art and future perspectives. JACC Cardiovasc Imaging. 2022;15(3):519–32.
PubMed Google Scholar
Kundu M, Ng JC, Awuah WA, Huang H, Yarlagadda R, Mehta A, et al. NeuroVerse: neurosurgery in the era of Metaverse and other technological breakthroughs. Postgrad Med J. 2023. https://doi.org/10.1093/postmj/qgad002 .
Article PubMed Google Scholar
Bruno RR, Bruining N, Jung C, Group V-IS. Virtual reality in intensive care. Intensive Care Med. 2022;48(9):1227–9.
Wolff G, Bruno RR, Reiter M, Kantzow B, Kelm M, Jung C. Virtual reality device training for extracorporeal membrane oxygenation. Crit Care. 2020;24(1):390.
Sutherland J, Belec J, Sheikh A, Chepelev L, Althobaity W, Chow BJW, et al. Applying modern virtual and augmented reality technologies to medical images and models. J Digit Imaging. 2019;32(1):38–53.
Puel F, Minville V, Vardon-Bounes F. What place for virtual reality in the intensive care unit during medical procedures? J Intensive Care. 2021;9(1):30.
Hoffman HG, Rodriguez RA, Gonzalez M, Bernardy M, Peña R, Beck W, et al. Immersive virtual reality as an adjunctive non-opioid analgesic for pre-dominantly Latin American children with large severe burn wounds during burn wound cleaning in the intensive care unit: a pilot study. Front Hum Neurosci. 2019;13:262.
Silva JNA, Southworth M, Raptis C, Silva J. Emerging applications of virtual reality in cardiovascular medicine. JACC Basic Transl Sci. 2018;3(3):420–30.
Page MJ, McKenzie JE, Bossuyt PM, Boutron I, Hoffmann TC, Mulrow CD, et al. The PRISMA 2020 statement: an updated guideline for reporting systematic reviews. BMJ. 2021;372: n71.
Nas J, Thannhauser J, Vart P, van Geuns RJ, Muijsers HEC, Mol JQ, et al. Effect of face-to-face vs virtual reality training on cardiopulmonary resuscitation quality: a randomized clinical trial. JAMA Cardiol. 2019. https://doi.org/10.1001/jamacardio.2019.4992 .
Article PubMed Central Google Scholar
Ricci S, Calandrino A, Borgonovo G, Chirico M, Casadio M. Viewpoint: virtual and augmented reality in basic and advanced life support training. JMIR Serious Games. 2022;10(1): e28595.
Colt HG, Crawford SW, Galbraith O 3rd. Virtual reality bronchoscopy simulation: a revolution in procedural training. Chest. 2001;120(4):1333–9.
CAS PubMed Google Scholar
Chiang DH, Huang CC, Cheng SC, Cheng JC, Wu CH, Huang SS, et al. Immersive virtual reality (VR) training increases the self-efficacy of in-hospital healthcare providers and patient families regarding tracheostomy-related knowledge and care skills: a prospective pre-post study. Medicine (Baltimore). 2022;101(2): e28570.
Ralston BH, Willett RC, Namperumal S, Brown NM, Walsh H, Muñoz RA, et al. Use of virtual reality for pediatric cardiac critical care simulation. Cureus. 2021;13(6): e15856.
Yu M, Yang M, Ku B, Mann JS. Effects of virtual reality simulation program regarding high-risk neonatal infection control on nursing students. Asian Nurs Res (Korean Soc Nurs Sci). 2021;15(3):189–96.
Yang SY, Oh YH. The effects of neonatal resuscitation gamification program using immersive virtual reality: a quasi-experimental study. Nurse Educ Today. 2022;117: 105464.
Farra S, Hodgson E, Miller ET, Timm N, Brady W, Gneuhs M, et al. Effects of virtual reality simulation on worker emergency evacuation of neonates. Disaster Med Public Health Prep. 2019;13(2):301–8.
Agasthya N, Penfil S, Slamon N. Virtual reality simulation for pediatric airway intubation readiness education. Cureus. 2020;12(12): e12059.
Nijland J, Veling W, Lestestuiver BP, Van Driel CMG. Virtual reality relaxation for reducing perceived stress of intensive care nurses during the COVID-19 pandemic. Front Psychol. 2021;12: 706527.
Bodet-Contentin L, Letourneur M, Ehrmann S. Virtual reality during work breaks to reduce fatigue of intensive unit caregivers: A crossover, pilot, randomised trial. Aust Crit Care. 2022. https://doi.org/10.1016/j.aucc.2022.01.009 .
Eccleston C, Crombez G. Pain demands attention: a cognitive-affective model of the interruptive function of pain. Psychol Bull. 1999;125(3):356–66.
Mosso-Vázquez JL, Gao K, Wiederhold BK, Wiederhold MD. Virtual reality for pain management in cardiac surgery. Cyberpsychol Behav Soc Netw. 2014;17(6):371–8.
Esumi R, Yokochi A, Shimaoka M, Kawamoto E. Virtual reality as a non-pharmacologic analgesic for fasciotomy wound infections in acute compartment syndrome: a case report. J Med Case Rep. 2020;14(1):46.
Laghlam D, Naudin C, Coroyer L, Aidan V, Malvy J, Rahoual G, et al. Virtual reality vs. Kalinox® for management of pain in intensive care unit after cardiac surgery: a randomized study. Ann Intensive Care. 2021;11(1):74.
CAS PubMed PubMed Central Google Scholar
Markus LA, Willems KE, Maruna CC, Schmitz CL, Pellino TA, Wish JR, et al. Virtual reality: feasibility of implementation in a regional burn center. Burns. 2009;35(7):967–9.
Hoffman HG, Patterson DR, Seibel E, Soltani M, Jewett-Leahy L, Sharar SR. Virtual reality pain control during burn wound debridement in the hydrotank. Clin J Pain. 2008;24(4):299–304.
Faber AW, Patterson DR, Bremer M. Repeated use of immersive virtual reality therapy to control pain during wound dressing changes in pediatric and adult burn patients. J Burn Care Res. 2013;34(5):563–8.
Merliot-Gailhoustet L, Raimbert C, Garnier O, Carr J, De Jong A, Molinari N, et al. Discomfort improvement for critically ill patients using electronic relaxation devices: results of the cross-over randomized controlled trial E-CHOISIR (Electronic-CHOIce of a SYSTEM for Intensive care Relaxation). Crit Care. 2022;26(1):263.
Haley AC, Wacker DA. Cinematic virtual reality for anxiety management in mechanically ventilated patients: a feasibility and pilot study. Acute Crit Care. 2022;37(2):230–6.
Lee SY, Kang J. Effect of virtual reality meditation on sleep quality of intensive care unit patients: a randomised controlled trial. Intensive Crit Care Nurs. 2020;59: 102849.
Squintani G, Brugnoli MP, Pasin E, Segatti A, Concon E, Polati E, et al. Changes in laser-evoked potentials during hypnotic analgesia for chronic pain: a pilot study. Ann Palliat Med. 2018;7(1):7–16.
Rousseaux F, Bicego A, Ledoux D, Massion P, Nyssen AS, Faymonville ME, et al. Hypnosis associated with 3D immersive virtual reality technology in the management of pain: a review of the literature. J Pain Res. 2020;13:1129–38.
Rousseaux F, Faymonville ME, Nyssen AS, Dardenne N, Ledoux D, Massion PB, et al. Can hypnosis and virtual reality reduce anxiety, pain and fatigue among patients who undergo cardiac surgery: a randomised controlled trial. Trials. 2020;21(1):330.
Rousseaux F, Dardenne N, Massion PB, Ledoux D, Bicego A, Donneau AF, et al. Virtual reality and hypnosis for anxiety and pain management in intensive care units: A prospective randomised trial among cardiac surgery patients. Eur J Anaesthesiol. 2021. https://doi.org/10.1097/EJA.0000000000001633 .
Jawed YT, Golovyan D, Lopez D, Khan SH, Wang S, Freund C, et al. Feasibility of a virtual reality intervention in the intensive care unit. Heart Lung. 2021;50(6):748–53.
Naef AC, Erne K, Exl MT, Nef T, Jeitziner MM. Visual and auditory stimulation for patients in the intensive care unit: a mixed-method study. Intensive Crit Care Nurs. 2022;73: 103306.
Suvajdzic M, Bihorac A, Rashidi P. D.R.E.A.M.S: (Digital rehabilitation environment-altering medical system). SeGAH IEEE Int Conf Serious Games Appl Health. 2017. https://doi.org/10.1109/SeGAH.2017.7939293 .
Article PubMed PubMed Central Google Scholar
Suvajdzic M, Bihorac A, Rashidi P, Ong T, Applebaum J. Virtual reality and human consciousness: the use of immersive environments in delirium therapy. Technoetic Arts. 2018;16(1):75–83.
Ong TL, Ruppert MM, Akbar M, Rashidi P, Ozrazgat-Baslanti T, Bihorac A, et al. Improving the intensive care patient experience with virtual reality-a feasibility study. Crit Care Explor. 2020;2(6): e0122.
Arofiati F, Primadani M, Ruhyana R. Role of family in the hospitalization of critical patients in the intensive care unit. Medisains. 2020. https://doi.org/10.30595/medisains.v18i1.6482 .
Article Google Scholar
He M, Li X, Zhang T, Jin X, Hu C. The fifth generation mobile communication technology plus virtual reality system for intensive care unit visits during COVID-19 pandemic: keep the delirium away. J Nurs Manag. 2022;30(8):3885–7.
Fiani B, Figueras RA, Samones P, Lee C, Castillo A, Sangalang B, et al. Long-Term Intensive Care Unit (ICU) Stays Can Lead to Long-Term Cognitive Impairment (LTCI): Neurosurgery Nursing Strategies to Minimize Risk. Cureus. 2022;14(9): e28967.
Turon M, Fernandez-Gonzalo S, Jodar M, Gomà G, Montanya J, Hernando D, et al. Feasibility and safety of virtual-reality-based early neurocognitive stimulation in critically ill patients. Ann Intensive Care. 2017;7(1):81.
Navarra-Ventura G, Gomà G, de Haro C, Jodar M, Sarlabous L, Hernando D, et al. Virtual reality-based early neurocognitive stimulation in critically Ill Patients: a pilot randomized clinical trial. J Pers Med. 2021;11(12):1260.
Marcarian T, Obreja V, Murray K, Meltzer JS, Miller PS. Success in supporting early mobility and exercise in a cardiothoracic intensive care unit. J Nurs Adm. 2023;53(3):161–7.
Gomes TT, Schujmann DS, Fu C. Rehabilitation through virtual reality: physical activity of patients admitted to the intensive care unit. Rev Bras Ter Intensiva. 2019;31(4):456–63.
Parke S, Hough CL, Aaron EB. The feasibility and acceptability of virtual therapy environments for early ICU mobilization. Pm r. 2020;12(12):1214–21.
Vlake JH, Van Bommel J, Wils EJ, Korevaar TIM, Hellemons ME, Schut AFC, et al. Effect of intensive care unit-specific virtual reality (ICU-VR) to improve psychological well-being and quality of life in COVID-19 ICU survivors: a study protocol for a multicentre, randomized controlled trial. Trials. 2021;22(1):328.
Vlake JH, Van Bommel J, Wils EJ, Korevaar TIM, Bienvenu OJ, Klijn E, et al. Virtual reality to improve sequelae of the postintensive care syndrome: a multicenter, randomized controlled feasibility study. Crit Care Explor. 2021;3(9): e0538.
Vlake JH, van Bommel J, Wils EJ, Bienvenu J, Hellemons ME, Korevaar TI, et al. Intensive care unit-specific virtual reality for critically Ill patients With COVID-19: multicenter randomized controlled trial. J Med Internet Res. 2022;24(1): e32368.
Vlake JH, van Bommel J, Hellemons ME, Wils EJ, Gommers D, van Genderen ME. Intensive care unit-specific virtual reality for psychological recovery after ICU treatment for COVID-19; a brief case report. Front Med (Lausanne). 2020;7: 629086.
Debelić I, Mikolčić A, Tihomirović J, Barić I, Lendić Đ, Nikšić Ž, et al. Stressful experiences of parents in the paediatric intensive care unit: searching for the most intensive PICU stressors. Int J Environ Res Public Health. 2022;19(18):11450.
Badke CM, Essner BS, O’Connell M, Malakooti MR. An innovative virtual reality experience in the PICU: a pilot study. Pediatr Crit Care Med. 2019;20(6):e283–6.
Badke CM, Krogh-Jespersen S, Flynn RM, Shukla A, Essner BS, Malakooti MR. Virtual reality in the pediatric intensive care unit: patient emotional and physiologic responses. Front Digit Health. 2022;4: 867961.
Kucher N, Larson JM, Fischer G, Mertaugh M, Peterson L, Gershan LA. 3-Dimensional nature-based therapeutics in pediatric patients with total pancreatectomy and islet auto-transplant. Complement Ther Med. 2020;48: 102249.
Abdulsatar F, Walker RG, Timmons BW, Choong K. “Wii-Hab” in critically ill children: a pilot trial. J Pediatr Rehabil Med. 2013;6(4):193–204.
Lai B, Powell M, Clement AG, Davis D, Swanson-Kimani E, Hayes L. Examining the feasibility of early mobilization with virtual reality gaming using head-mounted display and adaptive software with adolescents in the pediatric intensive care unit: case report. JMIR Rehabil Assist Technol. 2021;8(2): e28210.
Tallent S, Turi JL, Thompson J, Allareddy V, Hueckel R. Extending the radius of family-centered care in the pediatric cardiac intensive care unit through virtual rounding. J Am Assoc Nurse Pract. 2021;205–212. https://doi.org/10.1097/JXX.0000000000000610 .
Huang CY, Thomas JB, Alismail A, Cohen A, Almutairi W, Daher NS, et al. The use of augmented reality glasses in central line simulation: “see one, simulate many, do one competently, and teach everyone.” Adv Med Educ Pract. 2018;9:357–63.
Fumagalli S, Torricelli G, Massi M, Calvani S, Boni S, Roberts AT, et al. Effects of a new device to guide venous puncture in elderly critically ill patients: results of a pilot randomized study. Aging Clin Exp Res. 2017;29(2):335–9.
Morillas Perez J, Mecho Meca S, Caballero Galindo G, Miguel P-LJ. Validation of the effectiveness of augmented reality-assisted vascular puncture: an experimental model. J Vasc Access. 2023. https://doi.org/10.1177/11297298231156006 .
Alismail A, Thomas J, Daher NS, Cohen A, Almutairi W, Terry MH, et al. Augmented reality glasses improve adherence to evidence-based intubation practice. Adv Med Educ Pract. 2019;10:279–86.
Heo S, Moon S, Kim M, Park M, Cha WC, Son MH. An augmented reality-based guide for mechanical ventilator setup: prospective randomized pilot trial. jMIR Serious Games. 2022;10(3): e38433.
Gan A, Cohen A, Tan L. Augmented reality-assisted percutaneous dilatational tracheostomy in critically Ill patients with chronic respiratory disease. J Intensive Care Med. 2019;34(2):153–5.
Zackoff MW, Cruse B, Sahay RD, Fei L, Saupe J, Schwartz J, et al. Development and implementation of augmented reality enhanced high-fidelity simulation for recognition of patient decompensation. Simul Healthc. 2021;16(3):221–30.
Yamada Y, Nakamura T, Yamada M, Maruyama J, Maruyama K, Azuma E. Use of augmented reality to assist teaching for future perfusionists in extracorporeal technology. J Extra Corpor Technol. 2019;51(4):244–7.
Dias PL, Greenberg RG, Goldberg RN, Fisher K, Tanaka DT. Augmented reality-assisted video laryngoscopy and simulated neonatal intubations: a pilot study. Pediatrics. 2021. https://doi.org/10.1542/peds.2020-005009 .
Scquizzato T, Landoni G, Carenzo L, Forti A, Zangrillo A. A smartphone application with augmented reality for estimating weight in critically ill paediatric patients. Resuscitation. 2020;146:3–4.
Yeung AWK, Tosevska A, Klager E, Eibensteiner F, Laxar D, Stoyanov J, et al. Virtual and augmented reality applications in medicine: analysis of the scientific literature. J Med Internet Res. 2021;23(2): e25499.
Nas J, Thannhauser J, Vart P, van Geuns RJ, Muijsers HEC, Mol JQ, et al. Effect of face-to-face vs virtual reality training on cardiopulmonary resuscitation quality: a randomized clinical trial. JAMA Cardiol. 2020;5(3):328–35.
Gerber SM, Jeitziner MM, Wyss P, Chesham A, Urwyler P, Müri RM, et al. Visuo-acoustic stimulation that helps you to relax: a virtual reality setup for patients in the intensive care unit. Sci Rep. 2017;7(1):13228.
Hemphill S, Nguyen A, Kwong J, Rodriguez ST, Wang E, Caruso TJ. Virtual reality facilitates engagement in physical therapy in the pediatric CVICU. Pediatr Phys Ther. 2021;33(1):E7-e9.
Chillura A, Bramanti A, Tartamella F, Pisano MF, Clemente E, Lo Scrudato M, et al. Advances in the rehabilitation of intensive care unit acquired weakness: a case report on the promising use of robotics and virtual reality coupled to physiotherapy. Medicine (Baltimore). 2020;99(28): e20939.
OCEBM Levels of Evidence Working Group JH, Iain Chalmers (James Lind Library), Paul Glasziou, Trish Greenhalgh, Carl Heneghan, Alessandro Liberati, Ivan Moschetti, Bob Phillips, Hazel Thornton, Olive Goddard and Mary Hodgkinson. The Oxford Levels of Evidence 2. 2011.
Vlake JH, Wils EJ, van Bommel J, Korevaar TIM, Gommers D, van Genderen ME. Virtual reality tailored to the needs of post-ICU Patients: a safety and immersiveness study in healthy volunteers. Crit Care Explor. 2021;3(5): e0388.
Gerber SM, Jeitziner MM, Knobel SEJ, Mosimann UP, Müri RM, Jakob SM, et al. Perception and performance on a virtual reality cognitive stimulation for use in the intensive care unit: a non-randomized trial in critically Ill patients. Front Med (Lausanne). 2019;6:287.
Gerber SM, Jeitziner MM, Sanger SD, Knobel SEJ, Marchal-Crespo L, Muri RM, et al. Comparing the relaxing effects of different virtual reality environments in the intensive care unit: observational study. JMIR Perioper Med. 2019;2(2): e15579.
Vlake JH, van Genderen ME, Schut A, Verkade M, Wils EJ, Gommers D, et al. Patients suffering from psychological impairments following critical illness are in need of information. J Intensive Care. 2020;8:6.
Blair GJ, Kapil S, Cole SP, Rodriguez S. Virtual reality use in adult ICU to mitigate anxiety for a patient on V-V ECMO. J Clin Anesth. 2019;55:26–7.
Small C, Stone R, Pilsbury J, Bowden M, Bion J. Virtual restorative environment therapy as an adjunct to pain control during burn dressing changes: study protocol for a randomised controlled trial. Trials. 2015;16:329.
Naef AC, Jeitziner MM, Gerber SM, Jenni-Moser B, Muri RM, Jakob SM, et al. Virtual reality stimulation to reduce the incidence of delirium in critically ill patients: study protocol for a randomized clinical trial. Trials. 2021;22(1):174.
Umoren R, Bucher S, Hippe DS, Ezenwa BN, Fajolu IB, Okwako FM, et al. eHBB: a randomised controlled trial of virtual reality or video for neonatal resuscitation refresher training in healthcare workers in resource-scarce settings. BMJ Open. 2021;11(8): e048506.
Scapin SQ, Echevarría-Guanilo ME, Fuculo PRBJ, Martins JC, Barbosa MDV, Pereima MJL. Use of virtual reality for treating burned children: case reports. Rev Bras Enferm. 2017;70(6):1291–5.
Bloom D, Colombo JN, Miller N, Southworth MK, Andrews C, Henry A, et al. Early preclinical experience of a mixed reality ultrasound system with active GUIDance for NEedle-based interventions: the GUIDE study. Cardiovasc Digit Health J. 2022;3(5):232–40.
Download references
Acknowledgements
Not applicable.
Open Access funding enabled and organized by Projekt DEAL. This work was supported by the Forschungskommission of the Medical Faculty of the Heinrich-Heine-University Düsseldorf No. 2020-21 to RRB for a Clinician Scientist Track. Furthermore, institutional support has been received by the German Research Council (SFB 1116, B06) as well as the State of North Rhine Westphalia (Giga for Health: 5GMedizincampus. NRW, Project number 005-2008-0055 and PROFILNRW-2020-107-A, TP4). No (industry) sponsorship has been received.
Author information
Authors and affiliations.
Department of Cardiology, Pulmonology, and Vascular Medicine, Medical Faculty, Heinrich-Heine-University, University Hospital Duesseldorf, Duesseldorf, Germany
Dominika Kanschik, Raphael Romano Bruno, Georg Wolff, Malte Kelm & Christian Jung
Cardiovascular Research Institute Duesseldorf (CARID), Medical Faculty, Heinrich-Heine University, Duesseldorf, Duesseldorf, Germany
Malte Kelm & Christian Jung
You can also search for this author in PubMed Google Scholar
Contributions
DK and RRB performed a literature search, interpreted the patient data, and wrote the manuscript. GW, MK, and CJ reviewed the manuscript. All authors approved the final manuscript and assumed responsibility for the integrity of the data and the accuracy of the data analysis.
Corresponding author
Correspondence to Christian Jung .
Ethics declarations
Ethics approval and consent to participate.
Ethics approval was not applicable for the literature search in this study.
Consent for publication
The manuscript does not contain any individual person’s data in any form. People on images gave the written approval for the picture and its publication. VR glasses were not active on the images as shown and can be understand as examples. Local approval depending on relevant regulations needs to be obtained for the active use of such devices.
Competing interests
The authors declare that they have no competing interests.
Additional information
Publisher's note.
Springer Nature remains neutral with regard to jurisdictional claims in published maps and institutional affiliations.
See Table 3
Rights and permissions.
Open Access This article is licensed under a Creative Commons Attribution 4.0 International License, which permits use, sharing, adaptation, distribution and reproduction in any medium or format, as long as you give appropriate credit to the original author(s) and the source, provide a link to the Creative Commons licence, and indicate if changes were made. The images or other third party material in this article are included in the article's Creative Commons licence, unless indicated otherwise in a credit line to the material. If material is not included in the article's Creative Commons licence and your intended use is not permitted by statutory regulation or exceeds the permitted use, you will need to obtain permission directly from the copyright holder. To view a copy of this licence, visit http://creativecommons.org/licenses/by/4.0/ .
Reprints and permissions
About this article
Cite this article.
Kanschik, D., Bruno, R.R., Wolff, G. et al. Virtual and augmented reality in intensive care medicine: a systematic review. Ann. Intensive Care 13 , 81 (2023). https://doi.org/10.1186/s13613-023-01176-z
Download citation
Received : 05 May 2023
Accepted : 22 August 2023
Published : 11 September 2023
DOI : https://doi.org/10.1186/s13613-023-01176-z
Share this article
Anyone you share the following link with will be able to read this content:
Sorry, a shareable link is not currently available for this article.
Provided by the Springer Nature SharedIt content-sharing initiative
- Mixed reality
- Intensive care medicine
Advertisement
Trends in the Use of Augmented Reality, Virtual Reality, and Mixed Reality in Surgical Research: a Global Bibliometric and Visualized Analysis
- Review Article
- Published: 24 February 2022
- Volume 84 , pages 52–69, ( 2022 )
Cite this article
- Jing Zhang 1 na1 ,
- Na Yu 2 na1 ,
- Bin Wang 1 &
- Xin Lv ORCID: orcid.org/0000-0001-9611-7921 1
4853 Accesses
17 Citations
Explore all metrics
There have been many major developments in the use of augmented reality (AR), virtual reality (VR), and mixed reality (MR) technologies in the context of global surgical research, yet few reports on the trends in this field have been published to date. This study was therefore designed to explore these worldwide trends in this clinically important field. Relevant studies published from 1 January 2009 through 13 October 2020 were retrieved from the Science Citation Index-Expanded (SCI-E) tool of the Web of Science database. Bibliometric techniques were then used to analyze the resultant data, with visual bibliographic coupling, co-authorship, co-citation, co-occurrence, and publication trend analyses subsequently being conducted with GraphPad Prism 8 and with the visualization of similarities (VOS) software tool. There is no patient and public involved. In total, 6221 relevant studies were incorporated into this analysis. At a high level, clear global annual increases in the number of publications in this field were observed. The USA made the greatest contributions to this field over the studied period, with the highest H-index value, the most citations, and the greatest total link strength for analyzed publications. The country with the highest number of average citations per publication was Scotland. The Surgical Endoscopy And Other Interventional Techniques journal contributed the greatest number of publications in this field. The University of London was the institution that produced the greatest volume of research in this field. Overall, studies could be broadly classified into five clusters: Neurological Research, Surgical Techniques, Technological Products, Rehabilitative Medicine, and Clinical Therapy. The trends detected in the present analysis suggest that the number of global publications pertaining to the use of AR, VR, and MR techniques in surgical research is likely to increase in the coming years. Particular attention should be paid to emerging trends in related fields including MR, extended reality, head-mounted displays, navigation, and holographic images.
Similar content being viewed by others
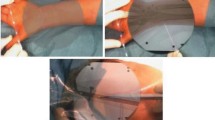
Clinical applications of augmented reality in orthopaedic surgery: a comprehensive narrative review
Johnathan R. Lex, Robert Koucheki, … David J. Backstein
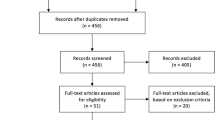
Applicability of augmented reality in orthopedic surgery – A systematic review
Lukas Jud, Javad Fotouhi, … Mazda Farshad
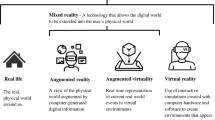
The impact of extended reality on surgery: a scoping review
James Zhang, Victor Lu & Vikas Khanduja
Avoid common mistakes on your manuscript.
Introduction
Virtual reality (VR) adds some substances and elements of the real world to the virtual space to make people feel the existence of the real world. Augmented reality (AR) adds a certain amount of virtual components and elements in the real space to make people feel that they have entered the virtual world. Mixed reality (MR) is the fusion of real space and virtual space, and artificial interaction in the real world and virtual world. The concept of VR first emerged in the 1960s, when Tom Furness developed technology that enabled jet pilots to access three-dimensional (3D) avionic data [ 1 ]. Many recent advances in VR and the related fields of AR and MR have been made in commercial contexts, with a range of VR systems harboring integrated haptic feedback peripherals having first been marketed to consumers in the 1980s and 1990s, although these platforms were expensive and suffered from relatively poor performance [ 2 ]. The limited commercial success of these VR/AR technologies led to their initial utilization primarily in academic or corporate contexts, leading to increased interest in their medical application [ 2 ]. The emergence of MR platforms has been particularly valuable in this space, as this hybrid of AR and VR technologies overcomes the respective limitations of these technologies by enabling users to interact with 3D data packets without excluding the surrounding real-world environment [ 3 ]. This hybrid MR approach thus enables the simultaneous manipulation of both real and virtual environments [ 4 , 5 ]. Sustained advances in the computing power underlying these altered reality-based technologies can subvert many healthcare-related fields, with surgery being a particularly promising context for the application of these novel tools [ 6 ]. Indeed, the combination of AR, VR, or MR techniques with robotic-assisted surgery has the potential to greatly improve surgical precision while decreasing operative durations and alleviating surgeon fatigue [ 3 ]. These technologies also offer clear value in contexts including medical education, doctor-patient communication, preoperative planning, intraoperative guidance and navigation, teleconsultation, and other surgery-related fields [ 7 ]. Consistent with such value, total spending on AR and VR products is forecast to exceed $215 billion in 2021, and the global healthcare AR and VR market is forecast to reach $5.1 billion by 2025 [ 2 ]. Many major medical and technological companies are thus seeking to develop novel approaches to combining surgical techniques and computer imaging modalities to achieve key breakthroughs, making a timely analysis of the application of VR, AR, and MR technologies in the field of surgical research of key importance. Few such studies have been published to date, highlighting the need to examine current and future trends in this research space in an effort to both understand and guide the directions of studies in this innovative and exciting field.
Peer-reviewed publications are a primary output associated with scientific research, and can be readily analyzed to gauge contributions to a given research field [ 8 ]. Bibliometric analyses and corresponding visual mapping techniques have been routinely utilized in recent years to assess scientific progress at a high level [ 9 ]. These analyses focus on the quantitative and qualitative evaluation of trends in a given research community over time based on data obtained from key online databases [ 10 ], comparing the relative contributions of different scholars, journals, institutions, and countries to a field of interest [ 11 ]. Bibliometric analyses have previously been leveraged to guide health policy and clinical guideline development, and to improve understanding of a range of conditions and technologies [ 12 ], including Middle East respiratory syndrome coronavirus (MERS-CoV) [ 13 ], exosomes [ 14 ], retinal regeneration [ 15 ], and spinal ultrasound [ 16 ]. The present study was therefore designed to assess current and future trends pertaining to the use of AR, VR, and MR technologies in the field of surgery and to identify emerging topics in this research space that are the focus of growing scholarly investigation.
For this analysis, VR was defined as a computer-generated 3D-simulated environment in which users are able to fully immerse themselves, while AR was defined as the projection of computer-generated images onto real-world entities, and MR was defined as the AR-like projection of virtual objects onto real-world entities such that these objects were responsive and spatially aware such that users were able to interact with these 3D projections [ 6 , 7 ] (Fig. 1 ).
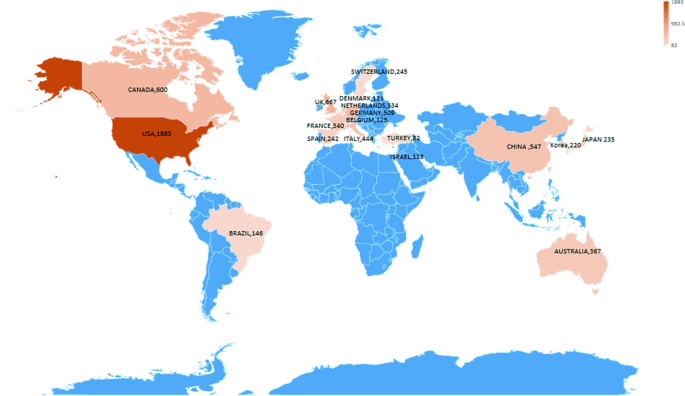
A map highlighting numbers of studies pertaining to the use of these technologies in surgery that have been published in different counties, with color depth corresponding to the numbers of publications per country
Materials and Methods
Data sources.
Bibliometric analyses were conducted by using the Science Citation Index-Expanded of the Web of Science (WOS), as this is the standard database used for such analyses [ 17 ]. No ethical oversight was required for this study, as it did not involve human subjects or preclinical animal models.
Search Strategy
The WOS database was searched for relevant studies published from January 2009 to October 2020 using the following search terms: (TS = “virtual reality” OR “augmented reality” OR “mixed reality”) AND (Language = English) AND (Document type = Articles OR Reviews). Key publication–related information including countries or regions of origin, institutions, funding sources, and research directions were then obtained from the WOS database. Studies were excluded from this analysis if they were unrelated to Surgery, including studies of Chemistry, Computer Theory, and Electrical Engineering.
Data Collection
Records for all studies identified through the above search strategy including title, year of publication, author names, institutional affiliations, nationality, keywords, abstracts, and publishing journals were saved in a.txt file format using the WOS and were opened in Microsoft Excel 2019 for analysis. Two co-authors (ZJ and YN) independently extracted data from these studies, with any discrepancies in extracted data being resolved through discussion or by contacting relevant experts.
Bibliometric Analysis
Bibliometric analyses leverage bibliometric theory to analyze relevant studies through statistical and mathematical approaches, thereby offering high-level insights into specific areas of scientific interest [ 18 , 19 ]. Intrinsic WOS tools can be used to assess the characteristics of studies included in bibliometric analyses. H-index values, for example, serve as key bibliometric indicators of scientific impact. H-values used to compute the H-index are based upon the number of times that publications from a given institution or researcher have been cited by other publications, thereby reflecting both the number of publications and the number of citations per published article [ 20 ]. The impact factor (IF) values for all journals in the present analysis were derived from the 2019 Journal Citation Reports.
Visualized Analysis
Past and predicted changes in publication volume over time were evaluated using a curve fitting model ( Y = AX − B ). Data pertaining to time-dependent trends in key bibliometric parameters were analyzed using GraphPad Prism (GraphPad Software Inc., CA, USA) [ 21 ]. VOS viewer (Leiden University, Leiden, Netherlands) was used for visual analyses of the bibliometric data and to conduct bibliographic coupling, co-authorship, co-citation, and co-occurrence analyses.
Global Publication Trends
Numbers of publications.
The number of scientific articles published in a particular field can offer key insight into current research trends in a given area. In total, we identified 6221 relevant studies in the WOS database published from 1 January 2009 to 13 October 2020 that met our search criteria, of which the majority (3559, 57.2%) were published within the last 5 years. We detected an overall upward trend in publications in this field over the past 12 years, suggesting that scholarly focus regarding the use of VR, AR, and MR in surgical contexts will continue to grow rapidly for the foreseeable future.
Country-Specific Contributions
The top 20 nations to have contributed to this field of research were next identified (Fig. 2 ). The greatest number of studies and reviews included in this analysis was published by research groups from the USA (1883, 30.27%), followed by those from England (677, 9.82%), Canada (600, 9.64%), Germany (509, 8.18%), and Italy 444, 7.14%) (Figs. 3 , 4 , and 5 ).
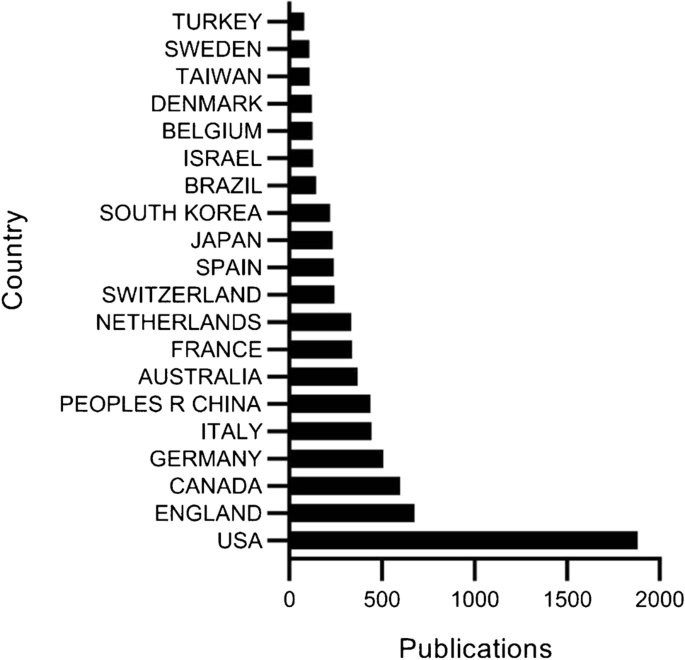
The total number of publications analyzing the use of AR, VR, and MR in surgical contexts from the top most prolific nations
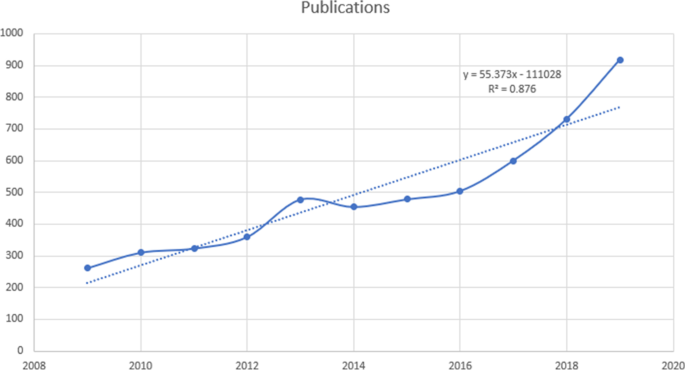
Model fitting curves were used to track trends in the future publication of studies pertaining to this topic of interest
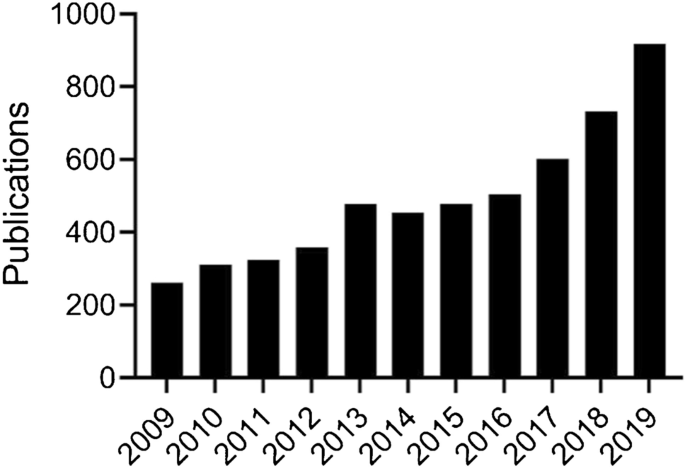
Annual numbers of publications related to the use of VR, AR, and MR in surgery published over the past 11 years. Institutional, research orientation, funding source, author, and journal contributions to this field of research
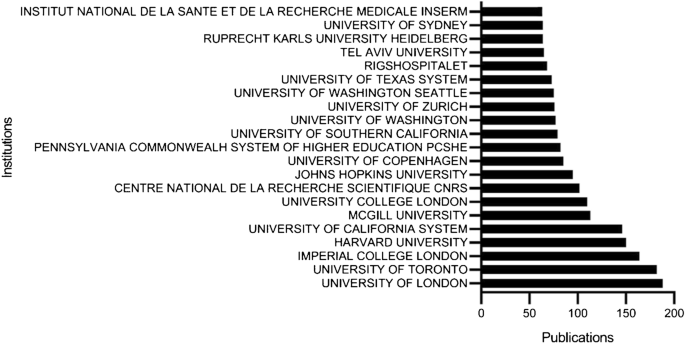
Institutions publishing the most studies pertaining to the use of AR, VR, and MR in surgery ranked according to number of publications
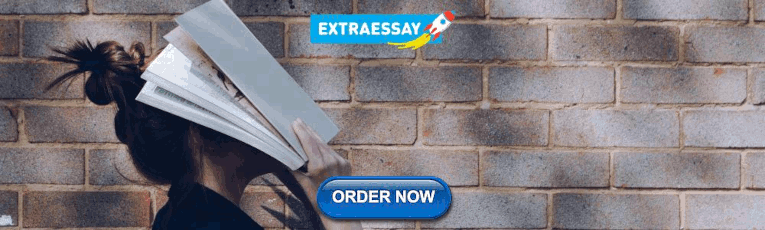
Institutional Contributions
The top 20 institutions and organizations to have engaged in research regarding the use of AR, VR, and MR in surgery are shown in Fig. 6 . Of these, the University of London has published the greatest number of studies (188), followed by the University of Toronto (44), and Imperial College London (35).
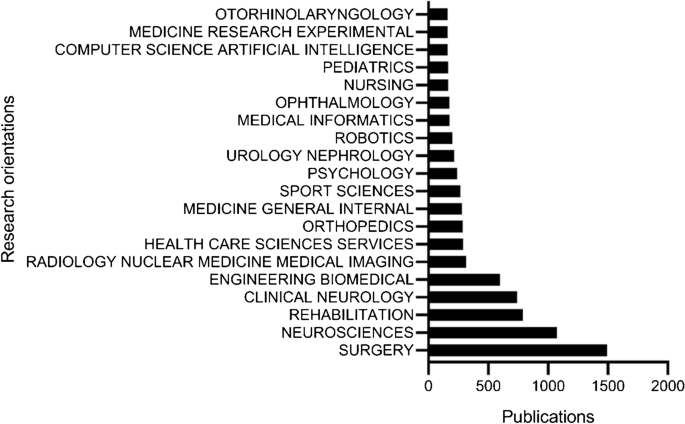
Research orientations publishing the most studies pertaining to this field of interest ranked according to numbers of publicationss
Contributions of Research Disciplines
The top three research areas that explored the use of AR, VR, and MR in surgery were the Surgery, Neuroscience, and Rehabilitation fields (Fig. 7 ).
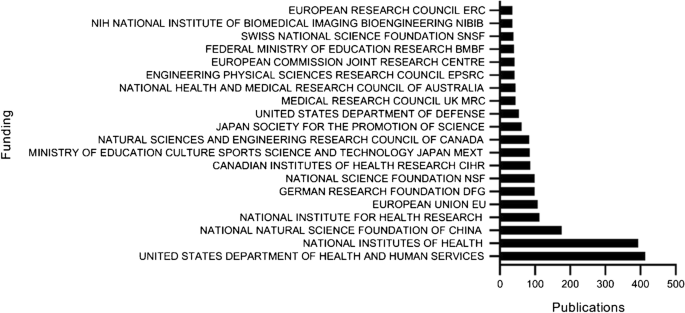
The most cited funding sources for studies pertaining to this field of interest ranked according to numbers of citing publications
Funding Source Contributions
The top 4 most commonly noted funding sources for studies included in this analysis were the US Department of Health and Human Services (413), the National Institutes of Health (NIH, USA) (394), the National Natural Science Foundation of China (175), and the National Institute for Health Research (NIHR)(112), as shown in Fig. 8 .
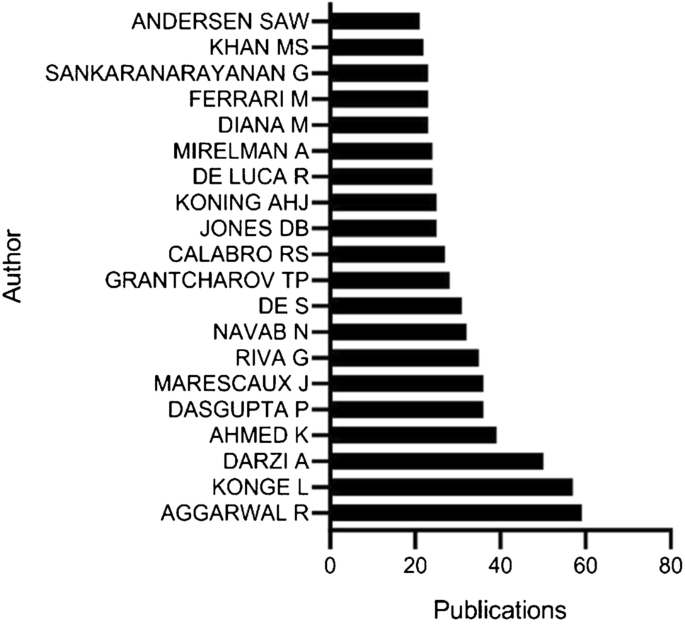
The authors publishing the most studies pertaining to this field of interest ranked according to numbers of publications
Contributions of Authors
The top 3 authors in this field were Aggarwal R, Konge L, and Darzi A, who respectively published 59, 57, and 50 articles in this research space (Fig. 9 ).
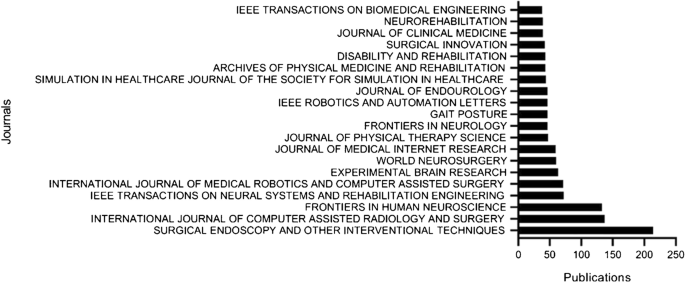
Journals publishing the most studies pertaining to this field of interest ranked according to numbers of publications. Citation frequencies and H-index values for individual nations
Journal Contributions
The top 5 journals publishing the most studies pertaining to the use of AR, VR, and MR in surgery identified in the present analysis were Surgical Endoscopy And Other Interventional Techniques ( n = 214), International Journal Of Computer Assisted Radiology And Surgery ( n = 137), Frontiers In Human Neuroscience ( n = 133), IEEE Transactions On Neural Systems And Rehabilitation Engineering ( n = 72), and the International Journal Of Medical Robotics And Computer Assisted Surgery ( n = 71) (Fig. 10 , Tables 1 and 2 ).
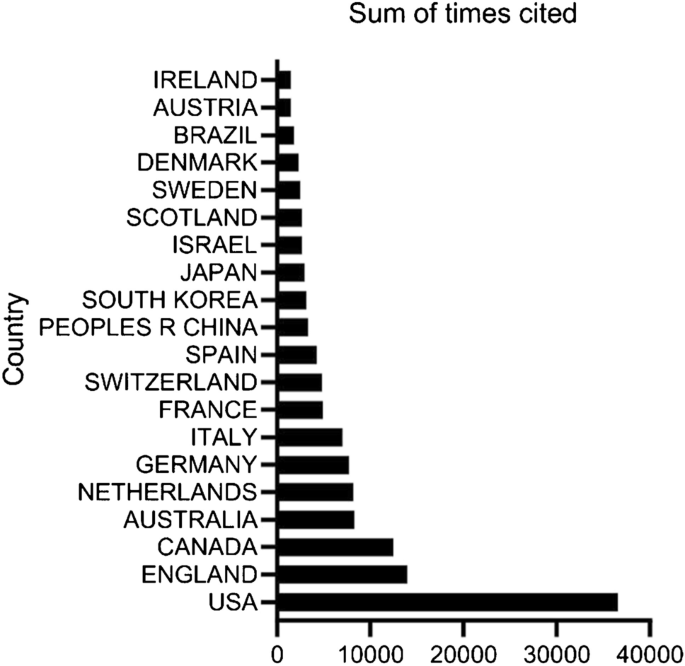
Total numbers of citations for studies pertaining to AR, VR, and MR use in surgery from the indicated countries
Quality of Publications from Individual Countries
Total citation frequency.
Studies from the USA had the greatest number of citations (36,577), followed by those from England (13,969), Canada (12,446), Australia (8290), and the Netherlands (8187) (Fig. 11 ).
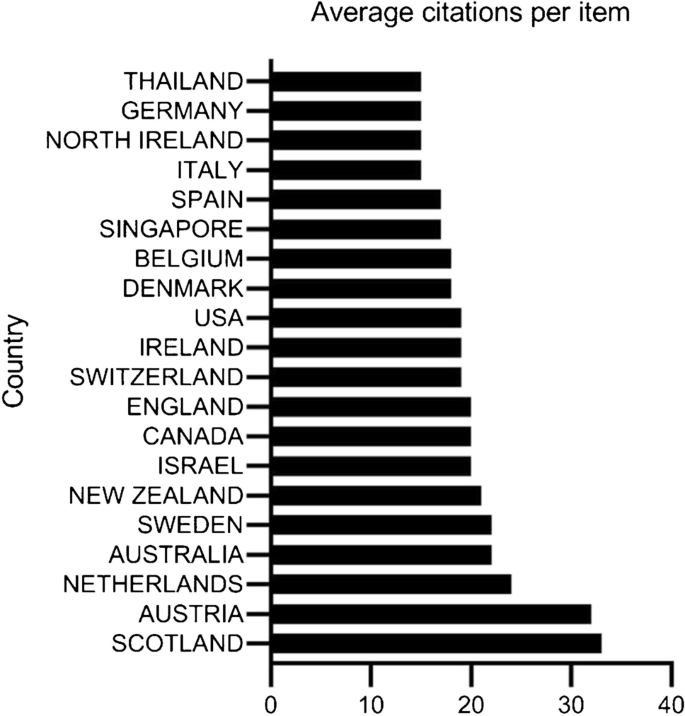
The average numbers of citations per publication for the indicated countries
Average Citation Frequency
Publications from Scotland exhibited the greatest average number of citations per study (33.08), followed by those from Austria (32.91), the Netherlands (80.74), Australia (22.59), and Sweden (22.52) (Fig. 12 ).
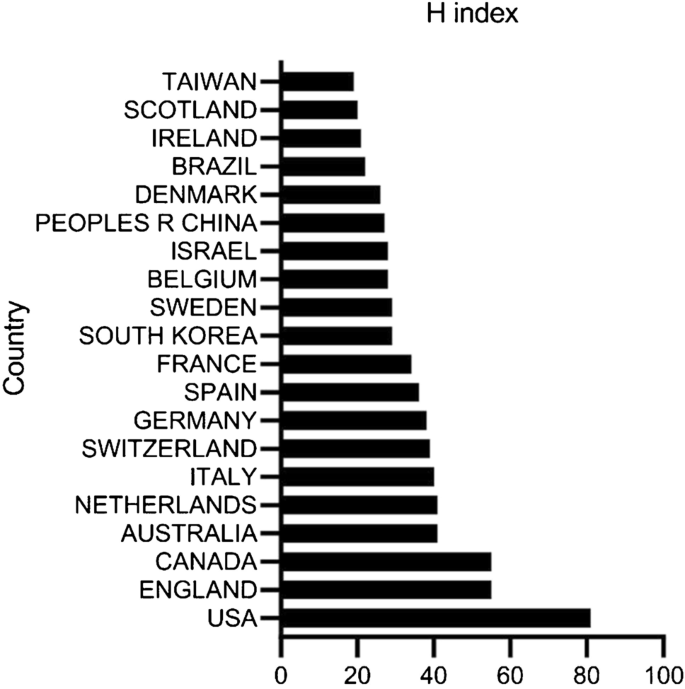
H-index values for publications from the indicated countries. Bibliographic coupling analysis of global research trends pertaining to studies of the use of AR, VR, and MR technologies in surgery.
H-Index Values
Publications included in this study from the USA had the highest H-index value (81), followed by those from England and Canada (55), Australia (41), the Netherlands (41), and Italy (40) (Fig. 13 ).
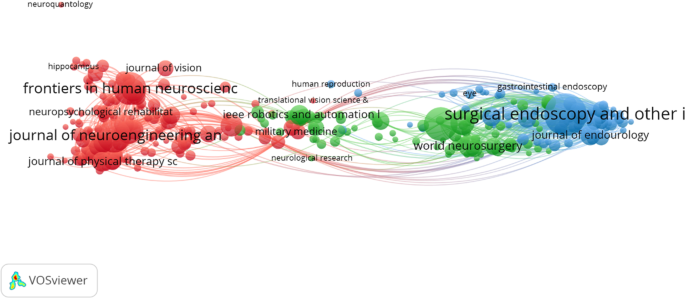
The mapping of 308 identified journals that have published studies in this research field
Bibliographic Coupling Analysis
Bibliographic coupling is a metric that can be used to detect similarity relationships between studies based upon their citations. When two studies both cite another study, this suggests that they share a related research focus. Bibliographic coupling analyses pertaining to journals, institutions, and countries were associated with relevant studies using VOS viewer, with individual spheres in the resultant mapping diagrams corresponding to the corresponding journals/institutions/countries, and with sphere size corresponding to total linkage strength (TLS) between individual elements, with greater numbers of links being indicative of stronger relationships between pairs of elements [ 22 ]. This approach can also be employed to conduct co-authorship, co-citation, and co-occurrence analyses.
The TLS for 308 journals publishing relevant articles pertaining to the use of VR, AR, and MR in surgery are shown in Fig. 14 . The journals with the greatest TLS values were Surgical Endoscopy And Other Interventional Techniques (Impact Factor, IF = 3.149, 2019; TLS = 88,700), Journal Of Neuroengineering and Rehabilitation (IF = 3.519, 2019; TLS = 48.242), Frontiers In Human Neuroscience (IF = 2.673, 2019; TLS = 30.797), American Journal of Surgery (IF = 2.125, 2019; TLS = 25,893), and Annals of Surgery (IF = 10.13, 2019; TLS = 25.739).
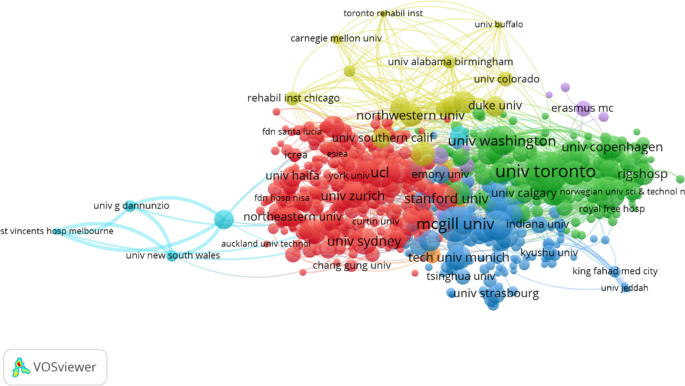
The mapping of 64 countries associated with publications in this field. Lines between two points indicate a relationship between the two connected items, with thicker lines corresponding to a closer link between these journals, institutions, or countries. Co-authorship analysis of global studies pertaining to the use of AR, VR, and MR in surgery
Institutions
Data from 676 institutions with 5 or more relevant publications were visualized using VOS viewer (Fig. 15 ), revealing the institutions with the greatest TLS values to be University of Toronto (TLS = 182,489); Imperial College of Science, Technology, and Medicine (TLS = 120,917); McGill University (TLS = 100,914); University of Washington (TLS = 83,718); and Technische Universiteit Delft (TLS = 60,754).
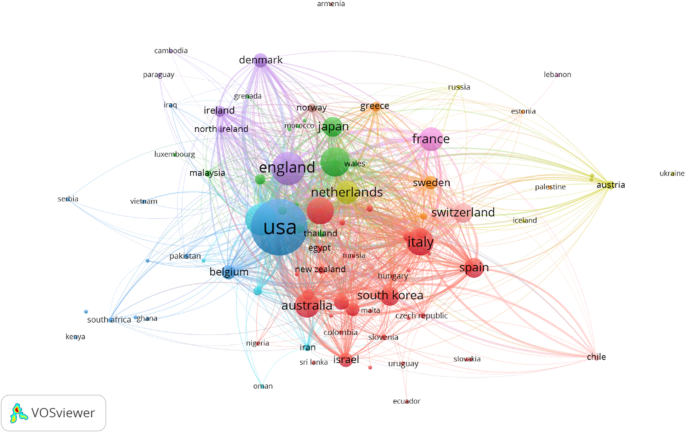
The mapping of 676 institutions that have published research in this field
Papers from 64 countries were assessed with VOS viewer (Fig. 16 ), revealing the countries with the greatest TLS values to be the USA (TLS = 686,681), England (TLS = 389,195), Canada (TLS = 343,385), Italy (TLS = 217,182), and Australia (TLS = 201,596).
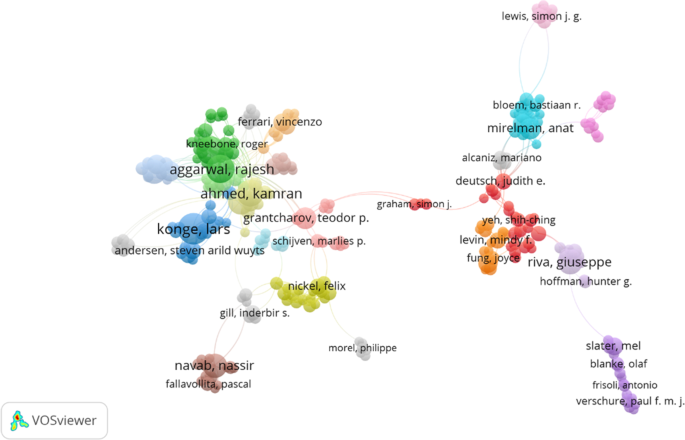
Co-authorship mapping for 581 authors associated with studies in this field of research
Co-authorship Analyses
Co-authorship analyses offer a means of assessing the relatedness of particular researchers or other items based upon numbers of co-authored publications, providing insights into collaboration among researchers, institutions, and countries in a given field of interest [ 21 ]. These analyses offer value as a means of providing researchers with valuable insights regarding potential collaborators, collaborative research group developments, and academic exchanges [ 22 ].
In total, we analyzed 581 different authors with a minimum of 5 publications using VOS viewer (Fig. 17 ). The authors with the greatest TLS were Calabro Rocco Salvatore (TLS = 133), De Luca Rosaria (TLS = 104), Ahmed Kamran (TLS = 94), Darzi Ara (TLS = 93), and Bramanti Placido (TLS = 89).
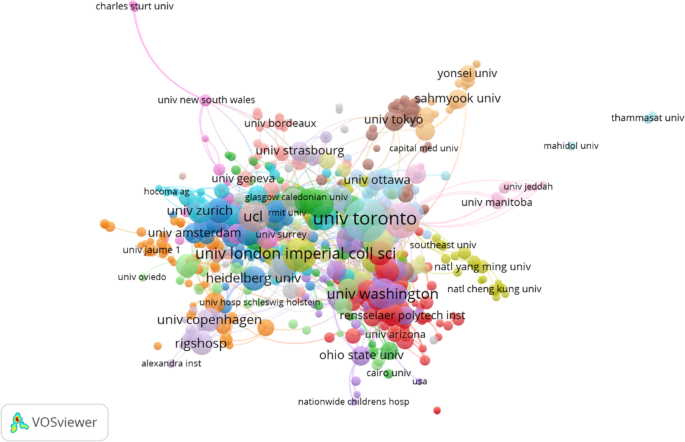
Co-authorship mapping was conducted for 676 institutions with studies in this field of research
In total, VOS viewer was used to analyze publications from 676 institutions (Fig. 18 ). The institutions with the greatest TLS values were the University of Toronto (TLS = 268), McGill University (TLS = 180), Harvard University (TLS = 138), Tel Aviv University (TLS = 129), and Johns Hopkins University (TLS = 128).
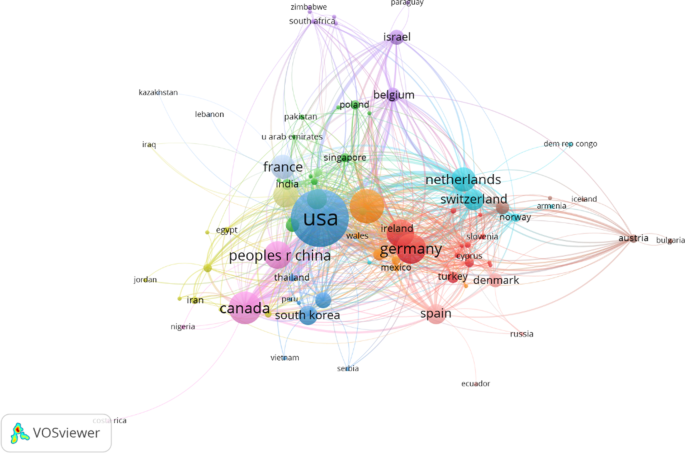
Co-authorship mapping was conducted for 99 countries with studies in this field of research. Point sizes correspond to co-authorship frequencies, with lines between points indicating an established collaboration between authors, institutions, or countries. Thicker lines correspond to closer collaborations between any two given authors, institutions, or countries. Co-citation analysis of global research trends pertaining to the use of AR, VR, and MR in surgery
In total, 99 different countries were analyzed with VOS viewer (Fig. 19 ). The countries with the greatest TLS values were the USA (TLS = 928), England (TLS = 645), Italy (TLS = 446), Germany (TLS = 445), and Canada (TLS = 418).
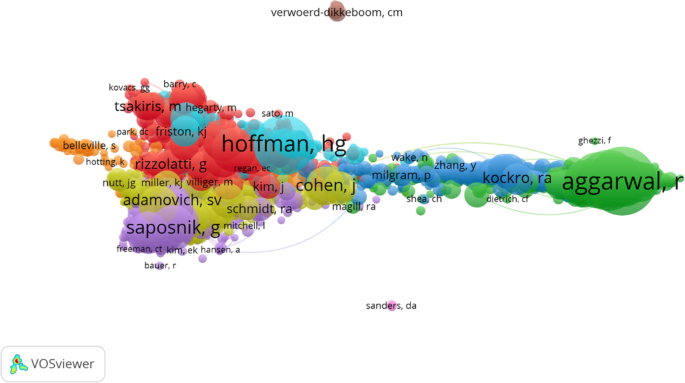
An author co-citation analysis was performed for studies in this research space, with points of a given color corresponding to shared research directions
Co-citation Analysis
Co-citation analyses are conducted by evaluating the number of times studies are cited together, thereby offering insights into correlations and similarities between articles and providing a firm knowledge base for a given field of interest.
In total, 922 authors with greater than 10 publications were next analyzed (Fig. 20 ), revealing the authors with the greatest TLS values to be Aggarwal R (TLS = 21.787), Gallagher AG (TLS = 15.005), Hoffman HG (TLS = 13.695), Grantcharov TP (TLS = 13.070), and Stefanidis D (TLS = 12.829).
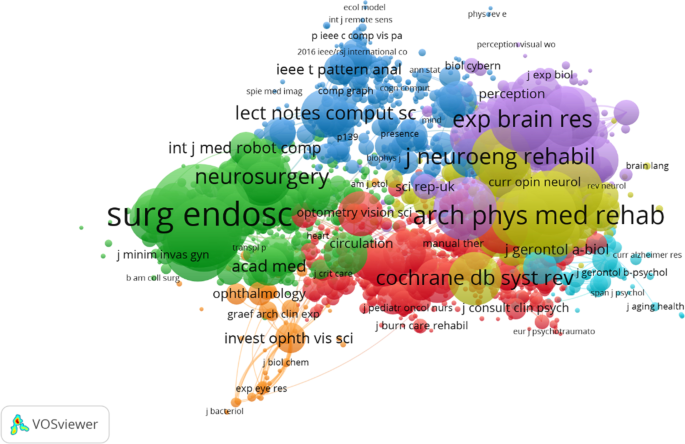
Co-cited journals in this research space were mapped, with point size corresponding to citation frequency. A line between two points indicates that both were cited in a given paper or journal, with shorter lines indicating a closer link between two given papers or journals. Co-occurrence analysis of global trends pertaining to the use of AR, VR, and MR technologies in the field of surgical research
Only journals with a least 20 citations were analyzed. The TLS values for these 1476 journals are shown in Fig. 21 . Among these top journals, the largest TLS values are Surgical Endoscopy And Other Interventional Techniques (TLS = 202,529), Archives Of Physical Medicine And Rehabilitation (TLS = 164,092), PLOS One (TLS = 147,507), International Journal Of Stroke (TLS = 131,524), and Neurorehabilitation And Neural Repair (TLS = 130,230).
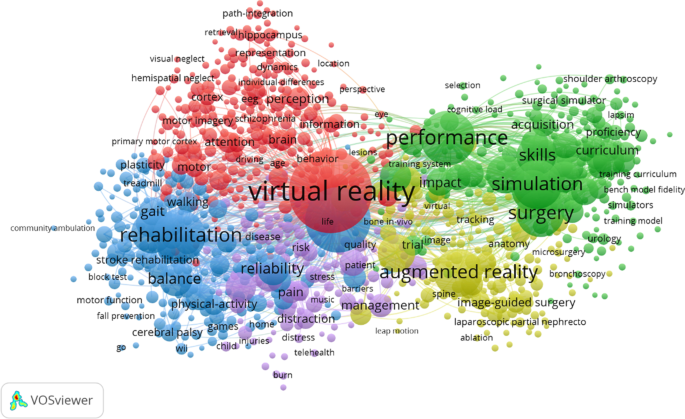
Keywords relevant to this analysis were mapped, with the size of individual points corresponding to frequency values. Overall, ;these keywords clustered into five categories: Neurological Research (red; upper left), Surgical Techniques (green; upper right), Technological Products (yellow; lower right), rehabilitative medicine (blue, lower left), and clinical therapy (purple, lower left)
Co-occurrence Analysis
Co-occurrence analyses offer a means of summarizing large numbers of research articles and predicting future trending topics in a given field, thereby elucidating and guiding scientific progress. To perform such a co-occurrence analysis, we used VOS viewer to visualize keywords used 10 or more times in the abstracts and titles of identified publications (Fig. 22 ). Overall, we identified 952 keywords that were clustered into five rough clusters pertaining to Neurological Research, Surgical Techniques, Technological Products, Rehabilitative Medicine, and Clinical Therapy, suggesting these to be the most prominent topics in this research field to date. The most frequently utilized keywords in the “Neurological Research” cluster included virtual reality, Alzheimer’s disease, Parkinson’s disease, brain, and movement. In the “Surgical Technique” cluster, the most commonly utilized keywords were performance, validation, simulation, surgery, and education, while in the “Technological Products” cluster, they were augmented reality, system, visualization, accuracy, and guidance. Similarly, the primary keywords for the “Rehabilitative Medicine” cluster were virtual reality, rehabilitation, balance, stroke, and exercise, while for the “Clinical Therapy” cluster, they were children, therapy, video games, distraction, and interventions.
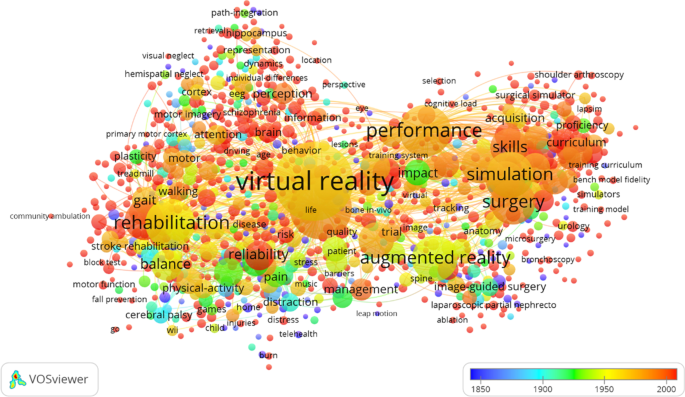
Keyword distributions based upon mean appearance frequency, with keywords in blue appearing earlier than keywords in red or yellow
VOS viewer was then used to color code these keywords based upon the frequency with which they appeared in the studies included in this analysis (Fig. 23 ), with blue indicating that keyword appeared at earlier time points and red indicating a later appearance. Prior to 2005, more studies focused on keywords including “Virtual Reality,” “Augmented Reality,” “Simulation,” “Rehabilitation,” and “Performance,” whereas rising trends suggest that “Mixed Reality,” “Extended Reality,” “Head-Mounted Display,” “Navigation,” and “Holographic Image” keywords are likely to be more frequently represented in future studies. A density visualization map was then generated for keywords included in this analysis, with the color corresponding to each keyword corresponding to the number of times it occurred in analyzed studies such that warmer colors indicate more frequent keyword utilization [ 23 ].
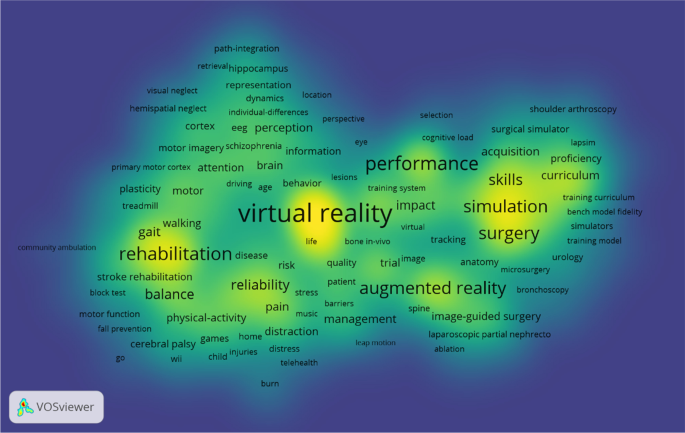
A density visualization map was generated based upon the times a given keyword occurred, which defined the area for a given term, with warmer colors corresponding to greater numbers of occurrences
Trends in VR, AR, and MR-Related Surgery Research
Bibliometric and visualized analyses offer opportunities to assess the current status of a given research field and to gauge future trends. There have been significant increases in the number of studies assessing the surgical applications of VR, AR, and MR over the last 5 years. Researchers from 99 different countries have published studies in this research space over the past decade, and our analysis suggests that this is an expanding area of interest that will be associated with the publication of rising numbers of high-quality articles in the coming years.
Global Publication Quality and Status
A global analysis of publications in this field revealed that the USA is responsible for the highest overall number of published papers, with the US Department of Health and Human Services being one of the most prominent funding sources in this research space. Perhaps unsurprisingly, surgical medicine was the most heavily researched branch of this field, and the USA exhibited the greatest contribution to this field with respect to total citations, H-index values, and publication numbers. England additionally exhibited the highest total citation counts of any countries contributing to this field, highlighting the USA and England as global leaders in the use of VR, AR, and MR in surgical medicine. Canada ranked third in terms of publication volume, H-index, and total citations in this field, while China was the sixth most published country but was only 16th with respect to the average number of citations per publication. This may be attributable to the historical emphasis on quantity rather than quality in the Chinese academic sector. However, the continued expansion of Chinese government-led medical research funding is likely to improve average study quality, helping China establish itself as a global research leader in this field.
When we conducted bibliographic coupling analyses of journals, institutions, and countries in this research space, we identified Surgical Endoscopy And Other Interventional Techniques , International Orthopaedics , International Journal Of Computer Assisted Radiology And Surgery , and Frontiers In Human Neuroscience as popular journals for the publication of studies evaluating the use of VR, AR, and MR in surgical contexts. Monitoring publications in these journals may thus be an effective means of tracking research trends in this field. We additionally found that the University of London was a leader in this field of research, while Imperial College London ranked third overall, consistent with the strong contributions of England to this research area. Harvard University and the University of California System were the top research institutions in the USA, while the University of Toronto in Canada ranked second in an institutional coupling analysis. These findings emphasize the close relationship between top-ranked universities and the overall academic level of a given country. We found that Aggarwal R, Konge L, and Darzi A were the researchers who have made the greatest contributions to this field, suggesting that monitoring their future publications will highlight key trends for future development in this space.
These analyses suggest that the University of Toronto, McGill University, and Harvard University represent optimal collaborative partners and co-authors for individuals conducting research on the use of VR, AR, and MR in surgery. Surgical Endoscopy And Other Interventional Techniques , Archives Of Physical Medicine And Rehabilitation , and PLOS One were the three most frequently cited journals in the field, while Aggarwal R, Gallagher AG, and Hoffman HG were the most highly cited and widely recognized researchers conducting these studies.
VR, AR, and MR Surgical Research Focus Areas
A co-occurrence network diagram generated herein highlighted five key research trends in this field: neurological research, surgical techniques, technological products, rehabilitative medicine, and clinical therapy.
At a more focused level, MR technologies have been deployed in the context of surgical education, enabling surgeons to more rapidly expand their skillsets to ensure career growth [ 24 ]. New AR, VR, and MR tools are also used with increasing frequency to ensure that patients and their families understand pertinent surgical procedures, reducing the potential for doctor-patient miscommunication [ 25 , 26 ]. Preoperative imaging technologies offer a clear view of the surgical site and surrounding tissues, enabling safer and more precise surgical planning [ 27 , 28 ], and VR, AR, and MR tools are therefore being used with increasing frequency in clinical surgery in disciplines such as neurosurgery, orthopedics, plastic surgery, otorhinolaryngology, thoracic surgery, and urology [ 29 , 30 ]. These approaches can reduce operative durations, radiation doses, and incision sizes, thereby providing patients with increasingly high-quality care. VR-based clinical rehabilitation platforms have also been built and applied in the field of orthopedics [ 31 ], while AR-based developments have improved teleconsultation quality and medical resource sharing [ 32 ].
At a high level, approaches to combining AR and 3D printing technologies appear to be a growing area of interest in surgical medicine [ 33 ], particularly in neurosurgery and orthopedics. At the same time, image-based surgical navigation strategies are being increasingly employed in the contexts of vascular surgery, brain pacemakers, and epilepsy electrode implantation [ 34 ]. AR-based head-mounted display (HMD) units leveraging holographic imaging technology are also being employed in top operating rooms throughout the world [ 35 ]. Growing trends suggest that the development of AR-based semi- or fully automated robots with integrated scanners and surgical arms is likely to dramatically influence the surgical field in the coming years [ 36 ]. Novel extended reality (XR) technologies incorporating elements of VR, AR, or MR are forecast to be used with rising frequency by surgeons as these tools undergo further development [ 3 , 37 ], and the integration of cloud-based services and healthcare data will ensure that these XR platforms are fully optimized to facilitate high-quality patient care. The results of this bibliometric analysis emphasize the crucial need for high-quality studies in this research space, highlighting key areas for additional experimental or clinical research and funding infusion as appropriate.
Colors in the generated density visualization map correspond to the frequencies of occurrence for particular keywords. This analysis suggested that Surgical Techniques and Technological Products are likely to be popular research subjects in this field, with many studies pertaining to extended reality, navigation, and holographic images having emerged in recent years.
Strengths and Limitations of This Study
By employing bibliometric and visualization techniques to highlight trends in the use of AR, VR, and MR technologies in the field of surgical research, we have herein provided a robust overview of this clinically important field. It is worth mentioning that, through the processing of GraphPad Prism and VOSviewer, the content and results of the above research can be presented to readers more intuitively and vividly.
Even so, there are certain limitations to our analyses. For one, we excluded studies not published in English, potentially introducing a language bias into our findings. In addition, we only analyzed studies published as of 13 October 2020, and our analyses thus fail to reflect more recent studies in this field. Future analyses should therefore be extended to the non-English literature to better understand global trends in this research field. At the same time, the literature we selected was all articles or reviews, and did not include conferences, books, and other forms, which would make our research results lack certain comprehensiveness. Similarly, when we searched, we selected the Web of Science database and did not search other databases, which would also lead to certain deviation in the inclusion of literature. We should avoid the above problems in the future research.
Bias Analysis
In the selection of research content, this paper did not include other research results other than treatises and reviews, and only English articles were selected instead of articles in other languages, and only the WOS database was referred to in the selection of database, so it was suspected of selection bias. Because the data extraction and statistics of the paper were completed by the two authors, the inherent investigator bias was suspected.
Conclusions
Overall, our study provides high-level overview of current trends in the application of AR, VR, and MR technologies in surgical research. While the USA remains the clear leader in this field, followed by England, Australia is actively growing as a major in this field that prioritizes academic quality and is thereby actively influencing associated scientific norms. Key areas of future research are forecast to include extended reality, navigation, and holographic image–related technologies. Through such analyses and the predicted overall expansion in the number of studies in this field, we predict that these technologies will continue to benefit medicine and to thereby improve the available quality of care for all patients.
Data Sharing
No additional data are available.
Abbreviations
Impact factor
Total link strength
Web of Science
Mertz L (2019) Virtual reality pioneer Tom Furness on the past, present, and future of VR in health care. IEEE Pulse 10(3):9–11. https://doi.org/10.1109/MPULS.2019.2911808
Sutherland J et al (2019) Applying modern virtual and augmented reality technologies to medical images and models. J Digit Imaging 32(1):38–53. https://doi.org/10.1007/s10278-018-0122-7
Sakai D et al (2020) Augmented, virtual and mixed reality in spinal surgery: a real-world experience. J Orthop Surg. https://doi.org/10.1177/2309499020952698
Saito Y et al (2020) Intraoperative 3D hologram support with mixed reality techniques in liver surgery. Ann Surg 271(1):e4–e7. https://doi.org/10.1097/SLA.0000000000003552
Yoshida S et al (2019) Mixed reality computed tomography-based surgical planning for partial nephrectomy using a head-mounted holographic computer. Int J Urol 26(6):681–682. https://doi.org/10.1111/iju.13954
Verhey JT, et al. (2020) Virtual, augmented, and mixed reality applications in orthopedic surgery. Int J Med Robot 16(2):e2067. https://doi.org/10.1002/rcs.2067
Hu HZ et al (2019) Application and prospect of mixed reality technology in medical field. Curr Med Sci 39(1):1–6. https://doi.org/10.1007/s11596-019-1992-8
Mao X, et al. (2020) The status and trends of coronavirus research: a global bibliometric and visualized analysis. Medicine 99(22):e20137. https://doi.org/10.1097/MD.0000000000020137
Tijssen RJ, Winnink J (2016) Twenty-first century macro-trends in the institutional fabric of science: bibliometric monitoring and analysis. Scientometrics 109(3):2181–2194. https://doi.org/10.1007/s11192-016-2041-z
Ekinci S et al (2015) Letter to the Editor regarding analysis of changing paradigms of management in 179 patients with spinal tuberculosis during a 12-year period and proposal of a new management algorithm. World Neurosurg 84(6):2072. https://doi.org/10.1016/j.wneu.2014.12.003
Pu QH, Lyu QL, Su HY (2016) Bibliometric analysis of scientific publications in transplantation journals from Mainland China, Japan, South Korea and Taiwan between 2006 and 2015. BMJ Open 6(8): p. e011623. https://doi.org/10.1136/bmjopen-2016-011623
Chien TW, et al. (2019) Choropleth map legend design for visualizing the most influential areas in article citation disparities: a bibliometric study. Medicine (Baltimore) 98(41): p. e17527. https://doi.org/10.1097/MD.0000000000017527
Zyoud SH (2016) Global research trends of Middle East respiratory syndrome coronavirus: a bibliometric analysis. BMC Infect Dis 16:255. https://doi.org/10.1186/s12879-016-1600-5
Wang B et al (2019) The state of exosomes research: a global visualized analysis. Biomed Res Int 2019:1495130. https://doi.org/10.1155/2019
Cislo-Pakuluk A, Marycz K (2017) A promising tool in retina regeneration: current perspectives and challenges when using mesenchymal progenitor stem cells in veterinary and human ophthalmological applications. Stem Cell Rev Rep 13(5):598–602. https://doi.org/10.1007/s12015-017-9750-4
Zhai X, et al. (2017) Global research trends in spinal ultrasound: a systematic bibliometric analysis. BMJ Open 7(10):e015317. https://doi.org/10.1136/bmjopen-2016-01531717
Aggarwal A et al (2016) The state of lung cancer research: a global analysis. J Thorac Oncol 11(7):1040–1050. https://doi.org/10.1016/j.jtho.2016.03.010
Pei W et al (2019) Research trends of acupuncture therapy on insomnia in two decades (from 1999 to 2018): a bibliometric analysis. BMC Complement Altern Med 19(1):225. https://doi.org/10.1186/s12906-019-2606-519
Zou X, Yue WL, Vu HL (2018) Visualization and analysis of mapping knowledge domain of road safety studies. Accid Anal Prev 118:131–145. https://doi.org/10.1016/j.aap.2018.06.010
Bertoli-Barsotti L, Lando T (2017) A theoretical model of the relationship between the h-index and other simple citation indicators. Scientometrics 111(3):1415–1448. https://doi.org/10.1007/s11192-017-2351-9
Mao X, et al. (2020) A global bibliometric and visualized analysis in the status and trends of subchondral bone research. Medicine (Baltimore) 99(22):e20406. https://doi.org/10.1097/MD.0000000000020406
Mao XJ, et al. (2020) The status and trends of coronavirus research: a global bibliometric and visualized analysis. Medicine. https://doi.org/10.1097/MD.0000000000020137
Zhai X, Wang Q, Li M (2016) Tu Youyou’s Nobel Prize and the academic evaluation system in China. The Lancet 387(10029). https://doi.org/10.1016/S0140-6736(16)30261-6
Stefan P et al (2018) Team training and assessment in mixed reality-based simulated operating room: current state of research in the field of simulation in spine surgery exemplified by the ATMEOS project. Unfallchirurg 121(4):271–277. https://doi.org/10.1007/s00113-018-0467-x
Wu X et al (2018) Mixed reality technology launches in orthopedic surgery for comprehensive preoperative management of complicated cervical fractures. Surg Innov 25(4):421–422. https://doi.org/10.1177/1553350618761758
Lu K et al (2018) Use of short message service and smartphone applications in the management of surgical patients: a systematic review. Telemed J E Health 24(6):406–414. https://doi.org/10.1089/tmj.2017.0123
Wu X et al (2018) Mixed reality technology-assisted orthopedics surgery navigation. Surg Innov 25(3):304–305. https://doi.org/10.1177/1553350618771413
Sauer IM et al (2017) Mixed reality in visceral surgery: development of a suitable workflow and evaluation of intraoperative use-cases. Ann Surg 266(5):706–712. https://doi.org/10.1097/SLA.0000000000002448
Incekara F et al (2018) Clinical feasibility of a wearable mixed-reality device in neurosurgery. World Neurosurg 118:e422–e427. https://doi.org/10.1016/j.wneu.2018.06.208
Lee SC et al (2017) Multi-modal imaging, model-based tracking, and mixed reality visualisation for orthopaedic surgery. Healthc Technol Lett 4(5):168–173. https://doi.org/10.1049/htl.2017.0066
Cabana F et al (2016) Is an in-home telerehabilitation program for people with proximal humerus fracture as effective as a conventional face-to face rehabilitation program? A study protocol for a noninferiority randomized clinical trial. BMC Sports Sci Med Rehabil 8(1):27. https://doi.org/10.1186/s13102-016-0051-z
Wang S, et al. (2017) Augmented reality as a telemedicine platform for remote procedural training. Sensors. https://doi.org/10.3390/s17102294
Negrillo-Cárdenas J, Jiménez-Pérez J-R, Feito FR (2020) The role of virtual and augmented reality in orthopedic trauma surgery: from diagnosis to rehabilitation. Computer Methods and Programs in Biomedicine. https://doi.org/10.1016/j.cmpb.2020.105407
Drouin S et al (2016) IBIS: an OR ready open-source platform for image-guided neurosurgery. Int J Comput Assist Radiol Surg 12(3):363–378. https://doi.org/10.1007/s11548-016-1478-0
Sayadi LR et al (2019) The new frontier: a review of augmented reality and virtual reality in plastic surgery. Aesthet Surg J 39(9):1007–1016. https://doi.org/10.1093/asj/sjz04336
Gorbanev I et al (2018) A systematic review of serious games in medical education: quality of evidence and pedagogical strategy. Med Educ Online 23(1):1438718. https://doi.org/10.1080/10872981.2018.1438718
Southworth MK, Silva JR, Silva JNA (2020) Use of extended realities in cardiology. Trends Cardiovasc Med 30(3):143–148. https://doi.org/10.1016/j.tcm.2019.04.005
Download references
This study was supported by a grant from National Nature Science Foundation of China (No. 81802204).
Author information
Jing Zhang and Na Yu contributed equally to this work.
Authors and Affiliations
Orthopedic Department, Second Hospital of Shanxi Medical University, Taiyuan, 030001, China
Jing Zhang, Bin Wang & Xin Lv
Cardiology Department, Second Hospital of Shanxi Medical University, Taiyuan, China
You can also search for this author in PubMed Google Scholar
Contributions
Xin Lv and Bin Wang conceived the research; Jing Zhang and Na Yu collected the data and prepared the manuscript; Jing Zhang and Na Yu analyzed the data; Na Yu provided techniques and advice.
Corresponding author
Correspondence to Xin Lv .
Ethics declarations
Ethics approval.
Ethical approval of the study was not necessary.
Competing Interests
The authors declare no competing interests.
Patient and Public Involvement
No patient involved. This review was not registered. The protocol was not prepared.
Additional information
Publisher's note.
Springer Nature remains neutral with regard to jurisdictional claims in published maps and institutional affiliations.
Rights and permissions
Reprints and permissions
About this article
Zhang, J., Yu, N., Wang, B. et al. Trends in the Use of Augmented Reality, Virtual Reality, and Mixed Reality in Surgical Research: a Global Bibliometric and Visualized Analysis. Indian J Surg 84 (Suppl 1), 52–69 (2022). https://doi.org/10.1007/s12262-021-03243-w
Download citation
Received : 14 May 2021
Accepted : 11 December 2021
Published : 24 February 2022
Issue Date : April 2022
DOI : https://doi.org/10.1007/s12262-021-03243-w
Share this article
Anyone you share the following link with will be able to read this content:
Sorry, a shareable link is not currently available for this article.
Provided by the Springer Nature SharedIt content-sharing initiative
- AR VR and MR
- Bibliometrics
- Visualized analysis
- Research trends
- Find a journal
- Publish with us
- Track your research
Login to your account
If you don't remember your password, you can reset it by entering your email address and clicking the Reset Password button. You will then receive an email that contains a secure link for resetting your password
If the address matches a valid account an email will be sent to __email__ with instructions for resetting your password
Access provided by

Download started.
- PDF [512 KB] PDF [512 KB]
- Figure Viewer
- Download Figures (PPT)
- Add To Online Library Powered By Mendeley
- Add To My Reading List
- Export Citation
- Create Citation Alert
Virtual reality applications toward medical field
Background/objectives, conclusions.
- 3D virtual data
- Virtual reality (VR)
1. Introduction
- Bouchard S.
- Dumoulin S.
- Robillard G.
- Scopus (202)
- Google Scholar
- Birckhead B.
- Scopus (165)
- Bordnick P.S.
- Carter B.L.
- Traylor A.C.
- Scopus (57)
- Scopus (33)
- Full Text PDF
- Scopus (152)
- Heyselaar E.
- Scopus (10)
- Merritt T.A.
- Parmentier B.L.
- Sharpton R.A.
- Takemoto J.K.
- Scopus (56)
- Scopus (55)
- Plahouras J.
- Johnston B.C.
- Scaffidi M.A.
- Grover S.C.
- Scopus (50)
- Mahrer N.E.
- Scopus (159)
- Scopus (44)
- Billinghurst M.
- Scopus (78)
- Scopus (22)
- Crossley R.
- Holtmannspoetter M.
- Scopus (26)
- Subramanian S.K.
- Lourenço C.B.
- Chilingaryan G.
- Sveistrup H.
- Scopus (147)
- Huygelier H.
- Schraepen B.
- VandenAbeele V.
- Gillebert C.R.
- Scopus (139)
2. What is virtual reality?
3. need for virtual reality in the medical field, 4. research objectives, 5. different benefits of virtual reality in the medical field.
- Gatica-Rojas V.
- Méndez-Rebolledo G.
- Scopus (64)
- Scopus (72)
- Porras D.C.
- Siemonsma P.
- Inzelberg R.
- Scopus (173)

- View Large Image
- Download Hi-res image
- Download (PPT)
- Scopus (32)
- Scopus (266)
6. Process of adoption of VR in the medical field
- Scopus (120)
- Paciorkowski A.R.
- Scopus (31)

7. Virtual reality applications in medical field
- Hamilton A.F.D.C.
- Scopus (347)
- Open table in a new tab
- Tataru C.A.
- Cojocaru C.M.
- Smolenski D.J.
- Tussyadiah I.P.
- tom-Dieck M.C.
- Scopus (561)
- Srivastava N.M.
- Nichterd M.
- Scopus (18)
- Puschmann P.
- Wittstock V.
- Scopus (114)
- Pizzoli S.F.M.
- Triberti S.
- Alcañiz Raya M.L.
- Pravettoni G.
- Scopus (59)
8. Discussion
9. limitations, 10. future scope, 11. conclusion, declaration of competing interest.
- Scopus (90)
- Diemer J.E.
- Alpers G.W.
- Peperkorn H.M.
- Mühlberger A.
- Scopus (553)
- Scopus (43)
- Garcia-Palacios A.
- Scopus (145)
- Scopus (186)
- Sarig-Bahat H.
- Williams K.
- Williams G.
- Treleaven J.
- Scopus (25)
- Scopus (96)
- HajesmaeelGohari S.
- NiakanKalhori S.R.
- Rothbaum B.O.
- Parsons T.D.
- Gaggioli A.
- Scopus (122)
- Beidel D.C.
- Scopus (94)
- Wichniarek R.
- Grajewski D.
- Zawadzki P.
- Scopus (121)
- ValmaggiaLR Latif L.
- Kempton M.J.
- Rus-Calafell M.
- Scopus (284)
- De Laurentiis M.
- Milanese C.
- Giordano A.
- Scopus (191)
- Alnajjar F.
- Alsinglawi B.
- Al Mahmud A.
- Scopus (66)
- Tashjian V.C.
- Mosadeghi S.
- Howard A.R.
- Samadbeik M.
- Yaaghobi D.
- Garavand A.
- Scopus (42)
- Maples-Keller J.L.
- Bunnell B.E.
- Scopus (414)
- Du Sert O.P.
- McIntosh K.S.
- Gregor J.C.
- Khanna N.V.
- Scopus (27)
- Linehan M.M.
- Hoffman H.G.
- Scopus (41)
- Sánchez-Cabrero R.
- Costa-Román Ó.
- Pericacho-Gómez F.J.
- Novillo-López M.Á.
- Arigita-García A.
- Barrientos-Fernández A.
- Scopus (19)
- Russell M.E.B.
- Stromberg S.
- Carlson C.R.
- Scopus (35)
- Scopus (412)
- De Domenico C.
- Scopus (16)
- Bergeron M.
- Lortie C.L.
- Guitton M.J.
- Scopus (67)
- Matamala-Gomez M.
- Bottiroli S.
- Sandrini G.
- Sanchez-Vives M.V.
- Tassorelli C.
- Scopus (113)
- Yildirim G.
- Yildirim S.
- Scopus (76)
- Scopus (24)
- Ventola C.L.
- Scopus (38)
- Scopus (102)
- Fanchiang H.D.
- Scopus (109)
Article info
Publication history, identification.
DOI: https://doi.org/10.1016/j.cegh.2019.12.010
User license

For non-commercial purposes:
- Read, print & download
- Redistribute or republish the final article
- Text & data mine
- Translate the article (private use only, not for distribution)
- Reuse portions or extracts from the article in other works
Not Permitted
- Sell or re-use for commercial purposes
- Distribute translations or adaptations of the article
ScienceDirect
- Download .PPT
Related Articles
- Access for Developing Countries
- Articles & Issues
- Articles In Press
- Current Issue
- Past Issues
- For Authors
- Author Information
- Researcher Academy
- Submit a Manuscript
- Journal Info
- About the Journal
- Contact Information
- Editorial Board
- New Content Alerts
The content on this site is intended for healthcare professionals.
- Privacy Policy
- Terms and Conditions
- Accessibility
- Help & Contact

SYSTEMATIC REVIEW article
Virtual reality and augmented reality in medical education: an umbrella review.
- 1 Department of Chemistry, Universidad Técnica Particular de Loja, Loja, Ecuador
- 2 Escuela Superior Politécnica de Chimborazo (ESPOCH), Riobamba, Ecuador
- 3 INFN-Laboratori Nazionali di Frascati, Frascati, Italy
Objective: This umbrella review aims to ascertain the extent to which immersive Virtual Reality (VR) and Augmented Reality (AR) technologies improve specific competencies in healthcare professionals within medical education and training, in contrast to traditional educational methods or no intervention.
Methods: Adhering to PRISMA guidelines and the PICOS approach, a systematic literature search was conducted across major databases to identify studies examining the use of VR and AR in medical education. Eligible studies were screened and categorized based on the PICOS criteria. Descriptive statistics and chi-square tests were employed to analyze the data, supplemented by the Fisher test for small sample sizes or specific conditions.
Analysis: The analysis involved cross-tabulating the stages of work (Development and Testing, Results, Evaluated) and variables of interest (Performance, Engagement, Performance and Engagement, Effectiveness, no evaluated) against the types of technologies used. Chi-square tests assessed the associations between these categorical variables.
Results: A total of 28 studies were included, with the majority reporting increased or positive effects from the use of immersive technologies. VR was the most frequently studied technology, particularly in the “Performance” and “Results” stages. The chi-square analysis, with a Pearson value close to significance ( p = 0.052), suggested a non-significant trend toward the association of VR with improved outcomes.
Conclusions: The results indicate that VR is a prevalent tool in the research landscape of medical education technologies, with a positive trend toward enhancing educational outcomes. However, the statistical analysis did not reveal a significant association, suggesting the need for further research with larger sample sizes. This review underscores the potential of immersive technologies to enhance medical training yet calls for more rigorous studies to establish definitive evidence of their efficacy.
1 Introduction
The advent of virtual reality (VR) and augmented reality (AR) technologies has initiated a paradigm shift in the domain of medical education and training. This umbrella review meticulously deconstructs and synthesizes the extensive contributions of these technologies, examining their potential to significantly elevate learner performance/engagement and improve pedagogical outcomes. Informed by the PICOS framework, our research question aims to assess the extent to which immersive VR and AR technologies, compared to traditional educational methods or no intervention, enhance specific competencies in healthcare professionals within medical education and training contexts.
In this context, the integration of haptic feedback technology, as investigated by Kapoor et al. ( 1 ), marks an important advancement in medical training. The tactile engagement provided by haptic interfaces in virtual environments has transformed the development of surgical skills, offering a high-fidelity replication of clinical procedures. Concurrently, AR technologies, as elucidated by Herron et al. ( 2 ) and Rochlen et al. ( 3 ), have begun to redefine the educational environment, merging interactive experiences with practical applications in a manner that traditional didactic methods cannot.
The deployment of VR in medical training, highlighted by Nguyen et al. ( 4 ), Childs et al. ( 5 ), and Walbron et al. ( 6 ), demonstrated the value of this technology as an educational asset. VR offers a diverse range of applications—from enhancing ophthalmologic examinations to simulating arthroscopic procedures—suggesting a significant shift from conventional training methods to those that are more cost-effective, repeatable, and learner-friendly.
The role of immersive technologies in fostering empathy within medical training is underscored by Elzie et al. ( 7 ), who demonstrated the effectiveness of VR in bridging the cognitive and emotional gap between healthcare providers and patients. This empathetic approach is complemented by the work of Gloy et al. ( 8 ) and Lian et al. ( 9 ), who attested the ability of VR to improve the retention of complex anatomical knowledge, indicating a marked advantage over traditional learning techniques in terms of engagement and information recall.
The importance of visual communication in healthcare, emphasized by Yaqi et al. ( 10 ), grants the potential of VR to elucidate complex medical conditions and treatments, thereby enhancing patient and provider experiences. This facet is integral to the educational transformation VR and AR are spearheading, as these technologies facilitate a deeper understanding and communication of medical concepts. Also, the development of VR training systems specifically designed for anatomy education and medical procedures, as explored by Falah et al. ( 11 ) and Ruthenbeck et al. ( 12 ), underlined the ability of these systems to improve learning outcomes and procedural competencies. Lee et al. ( 13 ) and Alfalah et al. ( 14 ) further illustrated practical applications of AR in enhancing the visualization of anatomical structures, an essential feature for surgical planning and educational purposes.
The study by Ivanov et al. ( 15 ) and Alahmadi et al. ( 16 ) signaled a leap forward in medical education through the application of AR in emergency medicine simulations and the development of educational apps like CardioSim, respectively. These innovations present engaging and effective methodologies for learning complex medical subjects.
Considering the disruptions caused by the COVID-19 pandemic, Dhar et al. ( 17 ) assessed the role of AR in sustaining the continuity of medical education, while Khundam et al. ( 18 ) contributed to the ongoing dialogue by examining different VR interaction modalities, affirming that technology can effectively accommodate diverse learning preferences without compromising outcomes. Albrecht et al. ( 19 ) advocated for the incorporation of VR simulators into medical curricula, reinforcing the acquisition of intricate clinical skills such as otoscopy.
The efficacy of AR in surgical oncology training and procedures is evaluated by Prunoiu et al. ( 20 ), highlighting AR's potential to enhance surgical performance. Toohey et al. ( 21 ) delved into the psychological impacts of simulation training, suggesting AR can create emotionally realistic yet manageable training scenarios. Finally, Lin et al. ( 22 ) and McBain et al. ( 23 ) demonstrate the capability of AR to advance complex professional skills and refine anatomical dissection training. In general, all these works delineate a trajectory toward an educational future where immersive technologies are not merely adjunct tools but are deeply integrated into the fabric of medical training, shaping the competencies of healthcare professionals in profound and multifaceted ways.
Hence, considering rapidly evolving technological sceneries and emerging global health challenges, this umbrella review aims to consolidate diverse perspectives and findings, providing an in-depth and comprehensive analysis of the role and future potential of VR and AR technologies in medical education—a domain of the cusp of significant transformation. Hence, adhering to PRISMA guidelines, our systematic review conducts a thorough literature synthesis to elucidate the effectiveness of immersive virtual and augmented reality technologies in enhancing medical training. Framed within the PICOS approach, our research question has been fashioned as:
• “To what extent do immersive virtual reality and augmented reality technologies, compared to traditional educational methods or no intervention, improve specific competencies in healthcare professionals as part of medical education and training?”
This question guides our exploration into the efficacy of VR and AR technologies in medical education, focusing specifically on outcomes like the retention of medical knowledge, the development of problem-solving skills, and the enhancement of critical thinking abilities. Our systematic review aims to provide a decisive answer to these pivotal questions, thus offering valuable insights into the transformative impact of VR and AR on the competence of medical professionals in an ever-evolving healthcare landscape.
2 Methodology
In this study, we utilize the PICOS approach, a rigorously established and widely acknowledged framework for formulating precise and focused research questions within systematic reviews ( 24 ). The PICOS model methodically breaks down the research question into five essential components, each serving to distill and clarify the key aspects of the inquiry. These components are succinctly presented in Table 1 , providing a structured overview of the research scope and direction, as follows:
• Population (P) has been specified to include both students and practicing healthcare professionals engaging in medical education and training.
• Intervention (I) mentions the use of VR and AR technologies in the educational process.
• Comparison (C) has been defined to include traditional educational methods or the lack of any intervention, which provides a baseline against which to measure the impact of VR and AR.
• Outcomes (O) have been detailed to reflect not just engagement or performance broadly, but specific competencies that are crucial in medical training.
• Study Design (S) has been expanded to include systematic reviews and meta-analyses, as these are the studies typically included in an umbrella review.
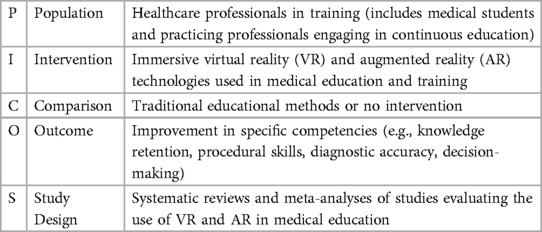
Table 1 . The research question of the presented review is defined according to the PICO approach.
This framework serves as a valuable tool for making precise research questions and devising study designs that facilitate the efficient collection of pertinent evidence.
Figure 1 delineates the methodological framework employed in our study, conforming to the PRISMA guidelines ( 25 ), and is characterized by a deliberate temporal boundary spanning from 2012 to 2022. This decade encapsulates a key period in the evolution and refinement of VR and AR technologies, marking significant milestones in their sophistication and integration into diverse domains. It is within this context that VR/AR technologies have seen substantial advancements in hardware and software capabilities, leading to enhanced realism and user experience. Moreover, this period is critical as it aligns with an increased adoption of these technologies in medical education and training, prompted by a growing recognition of their potential to enhance learning outcomes and simulate complex medical scenarios. Consequently, focusing on this transformative decade provides a robust understanding of the technological progression and educational implementation, offering a contemporary and relevant analysis of the impacts and advancements in the use of VR/AR in the medical field.
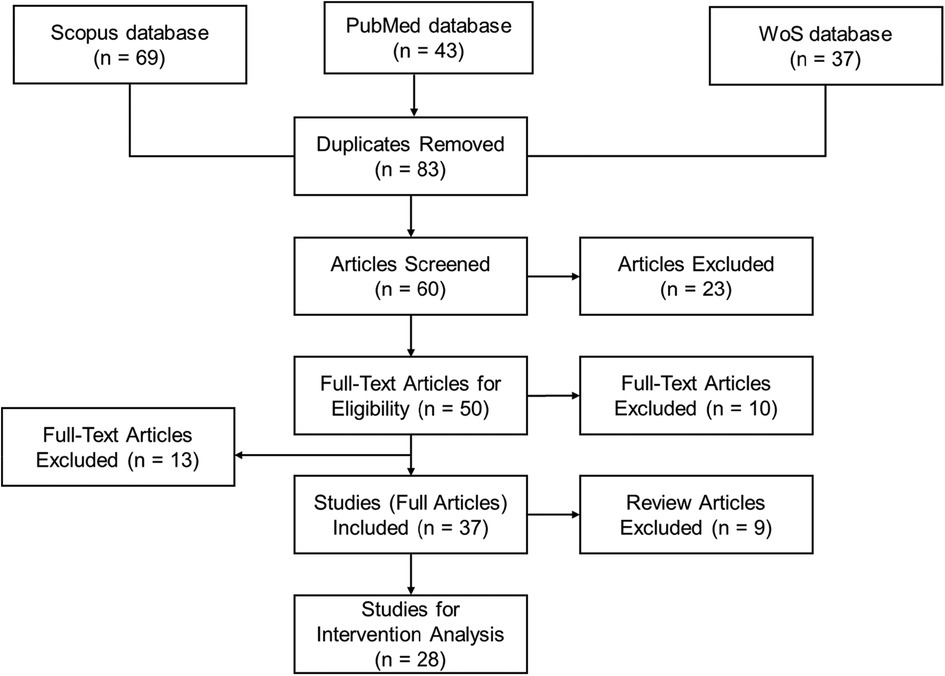
Figure 1 . Flow chart of current systematic review.
2.1 Identification
In May 2023, we executed a comprehensive search aimed at identifying pertinent literature for inclusion in this review. Our search strategy included three databases: Scopus, Web of Science, and PubMed. These platforms were selected for their extensive coverage, interdisciplinary content, and relevance to the medical and educational fields, thus ensuring a broad yet specific capture of literature about “ Exploration of Virtual Reality and Augmented Reality Applications in Medical Education and Training ”. Scopus offers a vast repository of peer-reviewed literature with a strong emphasis on scientific, technical, and medical content. Web of Science is known for its high-quality research articles and citation indexing, which facilitates the tracking of seminal work in the field. PubMed, a free resource developed by the National Center for Biotechnology Information (NCBI), is a premier database for biomedical literature from MEDLINE, life science journals, and online books.
These databases are preferred over other sources such as MEDLINE (partially indexed within PubMed and thus redundant), AMED (which focuses on allied health and complementary medicine, areas less central to our VR/AR focus), ERIC (which is education-focused but less comprehensive in medical training technologies), and CINAHL Plus (which concentrates on nursing and allied health, offering less scope in advanced medical education technologies). By harnessing the combined breadth and depth of Scopus, Web of Science, and PubMed, we ensure extensive coverage of the relevant literature without significant overlap, thereby streamlining our search process and maintaining a high standard of evidence selection.
These strategically targeted search results are summarized in Table 2 , which presents a synthesis of the article number retrieved during this phase.
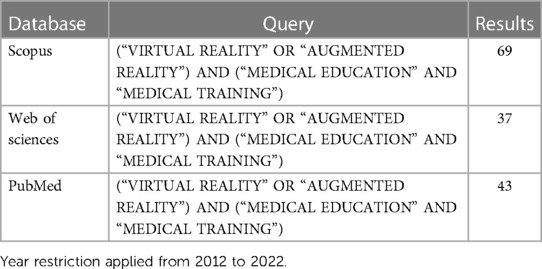
Table 2 . Query type and the corresponding results.
2.2 Screening
After conducting a comprehensive database search, we identified a total of 149 relevant articles: 69 from the Scopus database, 45 from PubMed, and 37 from the Web of Science. At this stage, we excluded conference papers, non-English language publications, books, and book chapters to focus exclusively on peer-reviewed journal articles. After the removal of duplicates, 83 unique articles remained. These articles were then subjected to a rigorous screening process where titles and abstracts were reviewed according to the following inclusion criteria, in line with our research question and the PRISMA guidelines:
• Inclusion of only full or original research articles, thereby excluding editorials, letters, and comments.
• Consideration of articles specifically about medical education and training, to ensure direct relevance to the focus of the current study.
• Requirement for the articles to discuss the use of VR, AR, or Immersive Virtual Reality technologies, reflecting our interest in these specific educational tools.
• Inclusion of articles that explore the application of simulators or learning outcomes in a medical context, aligning with the intervention component of our PICOS framework.
These criteria led to the exclusion of 23 articles during the screening stage. The remaining 60 articles were deemed suitable for full-text review to assess eligibility for inclusion in the final review. Each article was evaluated in detail to determine whether it conformed to our methodological and content-specific standards, which were pre-defined to minimize bias and enhance the systematic nature of the review process.
2.3 Eligibility
During the Eligibility phase, a systematic and unbiased assessment of the full texts was conducted. Each article from the screening phase was assigned randomly to members of the research team to ensure a thorough examination. We adhered to the following specific eligibility criteria, informed by the key concepts of our research question and study design:
• The article must explicitly discuss the application of AR and VR technologies within the context of medical education.
• The focus should be on immersive virtual reality as a tool for medical learning, teaching, or practical application.
• The implementation of a virtual environment must be primarily for educational purposes within the medical field.
During this full-text review, 50 articles satisfied all the eligibility criteria and were selected for detailed data extraction and subsequent analysis. Hence, we excluded 10 articles that did not demonstrate the application of a virtual environment for educational purposes in medicine. Additionally, 13 further articles were excluded as they lacked observable interventions related to medical education and training, which is a critical component of our review scope. Consequently, a total of 23 articles were deemed ineligible for inclusion in the data synthesis phase. However, given their relevance to the broader context of VR and AR use in medicine, these articles were instrumental in shaping the introduction section of our systematic review. They provided valuable background information and helped to delineate the background of current research in this field.
Therefore, 37 articles satisfied the inclusion criteria and subsequent analysis. This stage was critical in ensuring that our review was grounded in the most relevant and rigorous evidence available.
2.4 Included
In the final inclusion phase, we meticulously performed data extraction from the articles that fulfilled all our eligibility criteria. This process was designed to capture detailed information on several key aspects of the studies:
• The impact of the intervention on student performance and/or engagement.
• The specific type of virtual technology utilized in the intervention.
• The effects observed because of the intervention.
• The number of participants and their roles within the study.
As stated, we identified 37 articles that were directly aligned with our research objectives and thus included them for comprehensive analysis. The data extraction criteria focused on:
• The design of the virtual reality or augmented reality tool and the planning of its content.
• The development of learning materials within the virtual environment.
• The cognitive load imposed by virtual technology and associated time management.
• The feedback mechanisms and level of interactivity offered by the technology.
• The distribution of participants in the studies, categorized by their roles and involvement.
It is important to note that within this cohort, we identified 9 articles that were review papers. Consistent with the PRISMA guidelines, these were excluded from the main analysis because they did not present original research data. Nonetheless, these review articles were invaluable for contextual comparison and discussion in Section 4.3 of our systematic review. Their inclusion in this comparative discussion section helped to elucidate the novel contributions of our work to the existing body of knowledge. Therefore, a focused subset of 28 articles was advanced to the subsequent stage of in-depth intervention analysis, as detailed in the following sections of our review.
In our commitment to transparency and reproducibility, we have made our raw data, data processing details, and a summary of each stage of our review process publicly available. This information can be accessed at doi: 10.17605/OSF.IO/P3TGJ. This repository includes all relevant data and methodological steps, offering an open and comprehensive view of our research process.
3.1 Summary of search results
By the PRISMA guidelines and utilizing the PICOS approach, the results of the literature search and selection process for the systematic review on the impact of AR and VR in medical education and training are detailed in Figure 1 . Indeed, a comprehensive initial search across three databases—Scopus, PubMed, and Web of Science (WoS)—yielded a total of 149 articles, with 69, 43, and 37 articles identified from each respective database. After the removal of duplicate articles (i.e., 83 articles were considered at the initial stage), the remaining 60 articles were screened based on their titles and abstracts. Screening resulted in the exclusion of 23 articles that did not meet the predefined criteria. The remaining 50 articles underwent full-text review for eligibility, of which 13 were excluded. Reasons for exclusion at this phase included lack of relevance to the application of virtual environments for educational purposes, absence of interventions in the context of medical education and training, or the article type being a review rather than original research.
Therefore, 37 full articles were included for data extraction. These articles explored a range of interventions involving AR or VR technologies in medical education and training, assessing their effects on the performance of healthcare professionals, engagement, or both. At this point, 9 review articles were excluded from the intervention analysis but were utilized for contextual comparison in the systematic review, given their importance in framing the current state of AR and VR in medical education.
The remaining 28 studies were specifically selected for intervention analysis, which entailed a deeper investigation into the types of VR/AR interventions and their direct impact on learners within the medical field. The data extraction process focused on key factors such as the design and content planning of the tools, the development of learning materials, cognitive load management, feedback provided, interactivity of the simulations, and participant numbers based on roles.
To further emphasize, the selected 28 studies were evaluated in the context of the impact of immersive VR and AR interventions within medical education. These studies were particularly chosen for their relevance to our research question, as follows:
• The impact of these interventions on performance is meticulously defined and measured as an enhancement in the skill acquisition and knowledge retention of medical practitioners. This encompasses a broad spectrum of outcomes, including improved proficiency in various medical procedures, heightened accuracy in diagnostic techniques, and an overall increase in the retention of complex medical information. This dimension of performance is critical as it directly relates to the competencies that healthcare professionals must acquire and refine throughout their careers.
• Engagement, as evaluated in these studies, encompasses the multifaceted ways in which medical students interact with and embrace these emerging technologies. It is characterized by an increased willingness among students to integrate VR and AR systems into their learning paradigm, actively participate in technology-driven educational activities, and exhibit a sustained interest in the ongoing advancement of these immersive tools. Engagement is a crucial metric as it is indicative of both the acceptance of these technologies in the medical community and their potential to sustain long-term changes in medical education methodologies.
• The impact of interventions on both performance and engagement is believed to be fundamental in determining the efficacy of immersive technologies in medical education. These factors, when enhanced, suggest a substantial improvement in the quality of training for future healthcare professionals and a progressive shift towards more interactive, personalized, and effective learning experiences.
This systematic review, therefore, analyzes the quantifiable effects of immersive technologies on medical education. Table 3 shows the distinct impact of VR and AR technologies. Specifically, of the 28 studies scrutinized, 16 exclusively investigate the performance enhancements attributed to these technologies, while 3 focus solely on assessing learner engagement. A further 5 studies ambitiously evaluate both engagement and performance. Additionally, 1 study delves into the whole effectiveness of these interventions, and 3 studies scrutinize the applications of immersive virtual reality tools without a direct evaluation of outcomes.
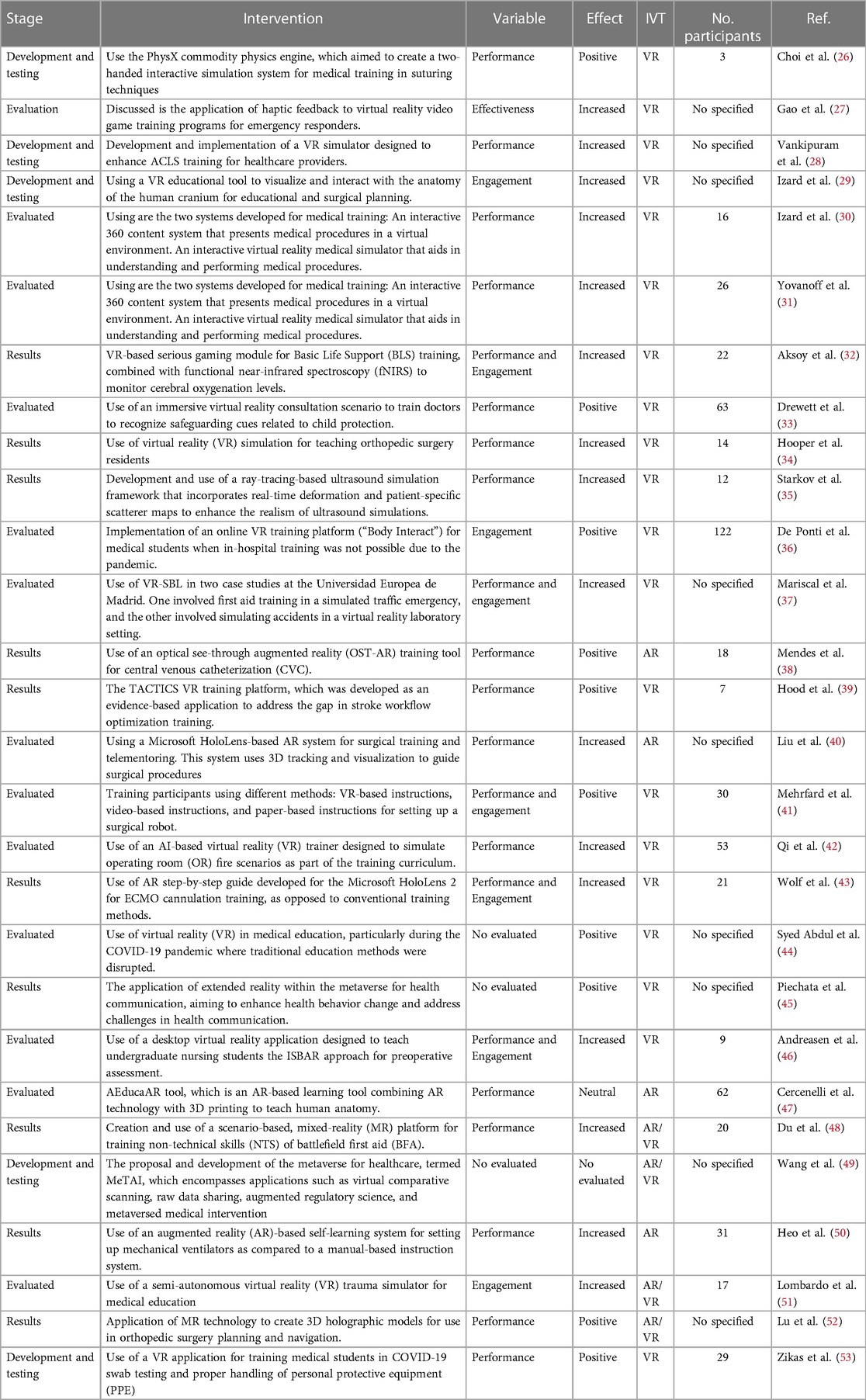
Table 3 . Extracted structural elements with corresponding interventions and measured effect.
The collective insights gleaned from this body of work underscore a predominant trend: an observable enhancement in educational outcomes linked to the integration of VR and AR in medical training. These interventions are consistently correlated with positive trends in both the acquisition of medical competencies and the active participation of students in their educational journeys. Nevertheless, there is an outlier; one study presents a neutral effect, which may stem from various factors that require further exploration to fully understand the implications of this finding on the broader application of immersive technologies in medical education. The nuances of this singular result serve as a reminder of the complexity inherent in educational interventions and the need for a multifaceted approach to their assessment and interpretation.
3.2 Summary of interventions
The synthesized data from Table 3 of the systematic review illustrates the outcomes of interventions involving VR and AR technologies in medical education, as delineated by distinct evaluative criteria:
• Regarding “Performance”, 16 studies were examined, revealing a predominantly positive impact. Of these, six reported a positive effect, one a neutral effect, and the remaining nine noted an increased effect on performance metrics. This suggests that the integration of VR and AR technologies in medical training has a generally favorable outcome on the performance capabilities of healthcare professionals.
• In the area of “Engagement”, three studies were evaluated. One of these studies observed a positive effect, while the other two reported an increased level of engagement among medical students and professionals. This enhancement in engagement indicates a promising shift towards more immersive and interactive learning environments facilitated by these technologies.
• For the combined metrics of “Performance and Engagement”, five studies were scrutinized, with one study indicating a positive effect and four showing an increased effect. This underlines the dual benefit of VR and AR technologies in simultaneously enhancing the learning process and the retention of knowledge and skills among medical trainees.
• One study focused on “Effectiveness”, which also reported an increased effect, reinforcing the notion that VR and AR interventions are not only engaging and performance-enhancing but also effective in their intended outcomes.
• Three studies were categorized as “No evaluated” due to their speculative or forward-looking nature. These studies are primarily perspective articles that, while not empirically evaluating outcomes, suggest potential positive effects of virtual technologies in two papers, with one work not assessing the effect.
These perspectives contribute to the broader understanding of the potential and future directions of immersive technologies in medical education, as follows:
• Choi et al. ( 26 ) developed a virtual suturing simulator that positively impacted medical training in suturing skills. This innovative tool integrated physics, graphics, and haptics simulation, improving user performance and offering a more efficient training method.
• Gao et al. ( 27 ) provided a case study that showed the effectiveness of haptic feedback in emergency responder training programs. This study demonstrated enhanced skill transfer to real-world situations for combat medics and civilian first responders, marking an advancement in medical simulation training.
• Vankipuram et al. ( 28 ) created an immersive VR simulator for Advanced Cardiac Life Support training. The simulator, which provided CPR feedback and recorded task-specific information, allowed for multi-user participation and remote learning, increasing the performance of healthcare providers in ACLS training.
• Hooper et al. ( 34 ) presented evidence of the effectiveness of VR simulation in improving orthopedic surgical skills of residents. This study showed that VR simulation could be a valuable addition to surgical education curricula, enhancing the training process for complex surgical procedures.
• Aksoy et al. ( 32 ) demonstrated the use of functional Near Infrared Spectroscopy (fNIRS) as a valuable tool for monitoring cognitive workload and assessing performance during VR-based Basic Life Support training. This integration improved learning outcomes and reduced cognitive workload, thus enhancing both performance and engagement in medical training.
Regarding the definition and application of VR in medical education, we admit the extreme inclusion of the study by Andreasen et al. ( 46 ). However, it is important to note that our systematic review adopts a comprehensive and inclusive approach to VR, recognizing that VR technology encompasses a spectrum of applications, from fully immersive environments to desktop simulations. The study by Andreasen et al., which evaluates a desktop-based VR application for preoperative ISBAR training, falls within this scope. This inclusion is supported by the fact that VR in education is an evolving field, with varied implementations that all serve the common goal of enhancing learning outcomes.
3.3 Data analysis
Figure 2 elucidates the distribution of studies examining the influence of VR and AR technologies on medical education across different variables. Performance, as a standalone metric, was the predominant focus of the studies, comprising 57.14% of the research. This prevalence underscores the criticality of evaluating the concrete skills and knowledge that learners acquire when engaged with immersive technologies. Engagement, reflecting the degree to which learners find the VR and AR experiences interactive and compelling, accounted for 17.86% of the studies, signifying a substantial interest in the user experience aspects of educational technology.
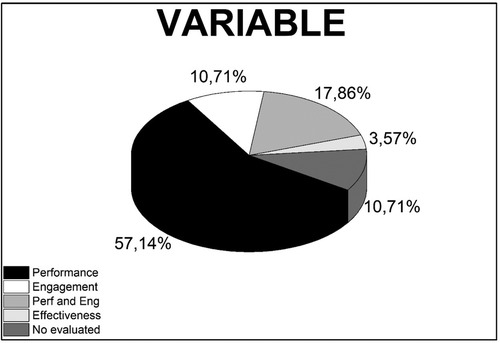
Figure 2 . Distribution of studies analyzing the variables on performance, engagement, and both performance and engagement (perf and Eng), effectiveness, and no evaluated in medical education utilizing VR and AR technologies.
A combined analysis of both performance and engagement was undertaken in 10.71% of the studies, reflecting an integrated approach to gauge the comprehensive effect of these technologies on learners—both in terms of skill acquisition and interactive participation. Effectiveness, potentially encompassing broader aspects such as the efficiency and practical utility of VR and AR, was also scrutinized in 10.71% of the studies, pointing to a concern for the general value and applicability of these technologies in medical education. The remaining 3.57% of studies did not assess these outcomes, which may suggest potential gaps in the current literature or deliberate exclusions where the impact of VR and AR was deemed not applicable or beyond the objectives of the respective studies. This fraction could represent an opportunity for future research to explore uncharted dimensions of VR and AR applications in medical training and education.
Figure 3 provides a visual breakdown of the observed effects from studies assessing the impact of VR and AR technologies in medical education. The pie chart reveals that a majority, 57.14% of studies, reported an ‘Increased’ effect, which signifies a substantial enhancement in the measured outcomes due to the application of these technologies. A “Positive” effect is reported in 35.71% of the studies, suggesting beneficial outcomes that, while perhaps not quantified, still indicate favorable changes attributable to the use of VR and AR. The “Neutral” category, accounting for 3.57% of the studies, implies that in these instances, VR and AR did not significantly alter the measured variables. Equally, at 3.57%, the “No evaluated” portion suggests that some studies did not measure or report specific outcomes, which could point to unexplored areas for future research or aspects that were outside the primary focus of the study.
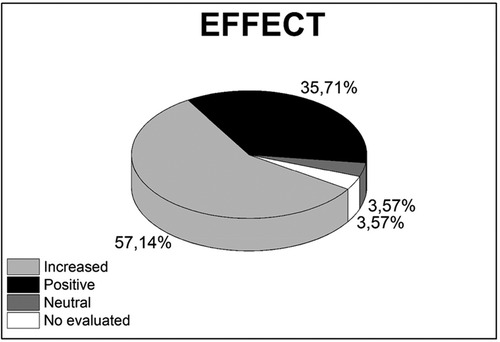
Figure 3 . Distribution of studies analyzing the effect on increase, positive, neutral, and No evaluated in medical education utilizing VR and AR technologies.
This distribution highlights the overwhelmingly positive reception of VR and AR technologies within medical education, with most studies recognizing their capacity to improve educational outcomes. The small percentage of studies with neutral or no evaluated effects indicates that while the general trend is towards benefit, there is room for a more nuanced exploration of the conditions under which these technologies may be most effective.
3.4 Descriptive analysis
The use of chi-square tests in the context of a systematic review is a methodological innovation that enhances the traditional narrative synthesis with a quantitative assessment of categorical data. While systematic reviews commonly aggregate findings qualitatively, the application of chi-square tests allows for a statistical exploration of the relationships between categorical variables such as the stage of work, variables studied, and effects observed in studies utilizing immersive virtual technologies.
In our systematic review, we elected to employ descriptive statistics to quantify the frequencies of various categories, as presented in Table 4 . The chi-square test is particularly suitable for this analysis as it determines whether there is a significant association between two categorical variables, which, in our case, are the stages, variables, and effects related to VR and AR technologies in medical education. When the expected frequencies are too low to meet the chi-square test assumptions, The Fisher test offers a more accurate assessment, thereby ensuring the robustness of our statistical analysis even with smaller sample sizes. Hence, the rationale for selecting a p -value of less than 0.05 is grounded in conventional statistical practice, which considers this level as the cutoff for rejecting the null hypothesis—that there is no association between the variables. By setting this threshold, we assert with 95% confidence that the observed relationships in our study are not merely the result of random variation but may reflect genuine correlations.
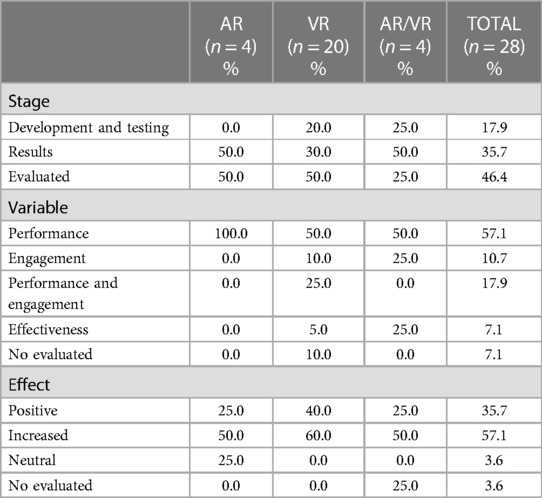
Table 4 . Prevalence of stage, variable, and effect in studies analyzed.
In the context of immersive virtual technologies versus Stage, we observe that:
• “Development and Testing” stage had 5 instances, with VR being the most used technology (4 times), followed by AR/VR (1 time), and AR was not used.
• In the “Results” stage, there were 10 instances. VR was again the most prevalent (6 times), followed by both AR and AR/VR being used twice.
• For the “Evaluated” stage, there were 13 instances where VR was used 10 times, indicating it is the most used technology in this stage, with AR and AR/VR being used less frequently (2 times for AR and once for AR/VR).
• General, VR was the most frequently reported technology (20 times), followed by AR (4 times) and AR/VR (4 times) across all stages.
• The Pearson Chi-square value is 1.982 with 4 degrees of freedom and a p -value of 0.739.
• The Likelihood ratio is 2.720 with 4 degrees of freedom and a p -value of 0.606.
• The Linear-by-Linear Association has a value of 0.859 with 1 degree of freedom and a p -value of 0.354.
Based on the chi-square test results, we conclude that there is no statistically significant relationship between the stages of work in medical education and the type of immersive technology used. VR appears to be the most utilized technology across all stages, but this usage does not statistically differ from the use of AR or combined AR/VR technologies in the context of the stages of work considered in this systematic review.
For immersive virtual technologies versus Variables, we point out that:
• The cross-tabulation displays the distribution of studies by the variable of interest (Performance, Engagement, Performance and Engagement, Effectiveness, No evaluated) against the type of immersive technology used (AR, VR, AR/VR).
• “Performance” is the most studied variable (16 instances in total), with VR being the most used technology (10 out of 16 times), followed by AR (4 times), and AR/VR (2 times).
• “Engagement” was studied less frequently (3 instances), with VR being used in 2 studies and AR/VR in 1 study. AR was not used for studies focusing solely on engagement.
• “Performance and Engagement” were jointly studied in 5 instances, with VR being used in all 5 studies.
• “Effectiveness” was evaluated in 2 instances, with both VR and AR/VR represented once.
• There were also 2 instances where the variable was “No evaluated,” with AR and VR each being used once.
• The Pearson Chi-square statistic is 7.700 with 8 degrees of freedom. The corresponding p -value is 0.463.
• The Likelihood Ratio statistic is 9.194 with 8 degrees of freedom. The p -value for this test is 0.326.
• The Linear-by-Linear Association statistic is 1.150 with 1 degree of freedom and a p -value of 0.284.
The distribution of studies according to the variable of interest and the type of immersive technology suggests a tendency towards VR in assessing both performance and engagement. However, the chi-square test indicates that these tendencies are not statistically significant. In essence, there appears to be no preferential association between the variables studied and the type of immersive technology used according to the sample of studies included in this review.
Finally, for immersive virtual technologies versus Effect, we show that:
• The cross-tabulation provides a breakdown of the effects (Positive, Increased, Neutral, No evaluated) observed in studies utilizing AR, VR, and AR/VR technologies.
• A “Positive” effect was reported in 10 instances, with VR having the highest frequency (8 times), followed by AR and AR/VR (1 time each).
• An “Increased” effect was the most common, with 16 instances. VR again had the highest frequency (12 times), AR had 2, and AR/VR also had 2.
• A “Neutral” effect was least common, with only one instance, which was associated with AR.
• There was 1 instance of “No evaluated” effect, which was associated with AR/VR.
• The Pearson Chi-square value is 12.460 with 6 degrees of freedom and a p -value of 0.052.
• The Likelihood Ratio value is 8.273 with 6 degrees of freedom and a p -value of 0.219.
• The Linear-by-Linear Association value is 0.255 with 1 degree of freedom and a p -value of 0.614.
The chi-square test results, particularly the Pearson Chi-square, suggest a potential pattern or trend in the data regarding the association between immersive technology type and observed effects, although this association does not reach conventional levels of statistical significance. There appears to be a stronger presence of “Increased” effects in studies involving VR, and “Positive” effects are also most frequently reported with VR. However, based on the current sample of studies, we cannot definitively conclude that there is a statistically significant association between the type of technology and the effect observed. Further research with larger sample sizes might be able to provide more definitive conclusions.
4 Discussions
4.1 intervention contributions.
It is important to focus on the diversity and impact of the interventions within the field of VR and AR in medical education, which are highlighted as follows:
• Choi et al. ( 26 ): This study represents a significant leap in medical training technology, particularly in suturing skills. The use of the PhysX physics engine to create a highly interactive simulation underscores the growing importance of realism in medical simulations. This work demonstrates the potential for VR to enhance fine motor skill development in medical professionals.
• Gao et al. ( 27 ): The application of haptic feedback in combat medic training is a pioneering contribution, particularly in enhancing the transfer of virtual training to real-world situations. This approach significantly improves the authenticity and effectiveness of training programs, potentially leading to better-prepared medics and first responders.
• Vankipuram et al. ( 28 ): The development of a VR ACLS simulator is notable for its emphasis on multi-user participation and remote learning capabilities. This study is a testament to the evolving nature of VR applications in medical training, where collaboration and distance learning are increasingly valued.
• Izard et al. ( 30 ): This research extends the utility of VR in medical education by allowing for repeated practice of surgical procedures. The development of interactive systems for understanding and performing medical procedures is a crucial step in automating surgical training and enhancing skill retention.
• Aksoy et al. ( 32 ): The integration of fNIRS technology for monitoring cognitive workload in VR-based training is a novel approach. This study contributes significantly to understanding how VR can be used to optimize learning by managing cognitive load, an aspect crucial for effective medical education.
• Mendes et al. ( 38 ): The validation of the OST-AR tool for CVC training represents an advancement in AR application in medical training. Its high acceptability and potential to enhance training without direct instructor intervention point to the future of autonomous learning tools in medical education.
• Wolf et al. ( 43 ): This exploration of AR in ECMO cannulation training illustrates the potential of this technology to standardize training and reduce errors in complex medical procedures. The emphasis on improving the clarity of information and stimulation during training marks a significant step forward in medical education technology.
These studies reflect the dynamic and evolving nature of VR and AR in medical education. They demonstrate how these technologies can enhance both the technical and cognitive aspects of medical training, from basic procedural skills to complex decision-making and problem-solving abilities. The integration of immersive technologies in medical education is not just about replicating real-world scenarios but also about enhancing and streamlining the learning process through innovative and interactive approaches.
4.2 Advantages
We have identified potential advantages of using immersive virtual technologies in medical education and training:
• Enhanced Realism and Immersion: Virtual technologies provide a highly immersive and interactive environment. For instance, Choi et al. ( 26 ) developed a virtual suturing simulator, and Izard et al. ( 29 ), a VR tool for studying human anatomy. Both works demonstrated how VR can create realistic settings for medical training, enhancing the understanding of complex procedures and anatomical structures.
• Improved Skill Transfer: The inclusion of haptic feedback in VR simulations, as explored by Gao et al. ( 27 ), aids in the effective transfer of skills learned in a virtual environment to real-world scenarios, which is critical in fields like combat medic training.
• Remote and Collaborative Learning: Virtual reality technology allows for remote learning and collaboration among medical trainees, breaking down geographical barriers and enabling a more inclusive and accessible education ( 28 ).
• Repeatable and Safe Practice: VR offers the opportunity for repeated practice in a risk-free environment. This is especially beneficial for procedures that cannot be frequently practiced on real patients ( 30 , 31 ).
• Cognitive Load Management: Tools like fNIRS integrated into VR training, as discussed by Aksoy et al. ( 32 ), enable monitoring and managing the cognitive workload of learners, ensuring that the training is both effective and not overwhelming.
• Innovative Training Methods: Augmented reality and AI integration in medical training, as seen in the works of Mendes et al. ( 38 ) and Qi et al. ( 42 ), offer new ways to train medical professionals, enhancing their learning experience with innovative approaches.
• Error Reduction and Standardization in Training: AR can reduce errors in complex medical scenarios and standardize training performance, as demonstrated by Wolf et al. ( 43 ) in ECMO cannulation training.
• Adaptability and Flexibility in Learning Environments: The flexibility of VR and AR technologies to adapt to various learning needs and scenarios, as highlighted in the works of Syed Abdul et al. ( 44 ) and Lombardo et al. ( 51 ), makes them invaluable tools in medical education.
• Enhanced Engagement and Motivation: The interactive nature of VR and AR, shown in studies like those of Mariscal et al. ( 37 ) and Cercenelli et al. ( 47 ), fosters greater student engagement and motivation, leading to potentially better learning outcomes.
These advantages demonstrate the substantial impact that virtual technologies can have in revolutionizing medical education, making learning more interactive, effective, and adaptable to various needs and scenarios.
4.3 Comparison with previous reviews
Our systematic review, as delineated in Table 5 (This Review), advances the discourse on immersive technologies within the realm of medical education by offering a complete examination that includes both AR and VR. Diverging from prior reviews ( 54 – 62 ) that may have concentrated on singular technology or a broader educational purview, our review distinctively provides a side-by-side evaluation of the influence of AR and VR on performance (P) and engagement (E)—critical elements for efficacious learning. These analyses are particularly salient, delving into the adaptability of these technologies to fulfill the unique pedagogical requirements and learning targets specific to medical training.
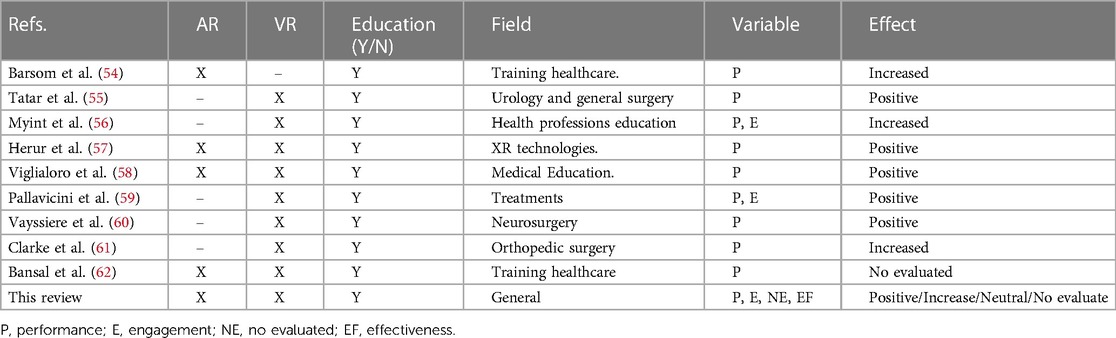
Table 5 . Comparison with previous review papers.
Our systematic review rises above a general assessment of technological efficacy, instead focusing on attributes such as performance optimization and engagement deepening, along with their resultant impacts. Such a focus is indispensable in medical education, where the cultivation of practical skills and sustained engagement are essential for the realization of favorable educational outcomes.
4.4 Restrictions
This systematic review admits certain constraints inherent to the scope and methodology of academic research. The burgeoning quantity of literature on VR and AR within medical education presented a challenge, raising the possibility of inadvertently omitting relevant studies. The meticulous crafting of search queries and selection of keywords was decisive to the comprehensiveness. While the “snowballing” method was instrumental in uncovering pertinent keywords and studies, significant contributions may have been missed due to the imposed limitations and time constraints inherent in the research process.
Additionally, the review was bound by selective inclusion criteria, particularly the focus on peer-reviewed journal articles published in English. This restriction may have led to the exclusion of seminal research conducted in other languages or significant findings disseminated through non-journal outlets, potentially skewing the representation of the field's international breadth and diversity. To surmount these limitations and cultivate a richer synthesis of knowledge, subsequent inquiries should contemplate an expansion of the inclusion parameters. Incorporating studies in multiple languages and integrating grey literature, such as conference proceedings, theses, and technical reports, could yield a more holistic panorama of the worldwide progress and deployment of AR/VR technologies in medical education. Such a widened scope would not only enhance the universality of the systematic review's findings but also provide a more inclusive map of the field's innovation and practice.
4.5 Recommendations for future research
The adoption of VR and AR in medical education calls for a globally recognized framework that delineates essential elements of VR and AR applications within a learner-centric pedagogical model. This framework should articulate core standards and a cohesive understanding of their implementation in medical pedagogy. This framework must maintain flexibility to accommodate ongoing technological progress and emerging insights from educational psychology.
Current research paves the way for an in-depth exploration into the seamless incorporation of these innovative teaching methodologies into the fabric of VR/AR-enhanced medical education. Future studies should endeavor to elucidate how VR and AR technologies can not only heighten student engagement but also facilitate customized learning journeys, tailoring educational experiences to cater to the diverse needs and learning preferences of individual students.
As VR and AR technologies become more entrenched in the educational landscape, it is crucial to navigate the accompanying ethical considerations and privacy concerns. Subsequent research should rigorously examine these aspects, with a particular focus on the ethical handling of simulated patient data and the implications of using such technologies in student evaluation and training. It is essential to ensure that VR and AR integration into medical education adheres to ethical norms and maintains the sanctity of confidentiality.
Moreover, a meticulous evaluation of the array of VR and AR applications currently employed in medical education is vital. Such assessments should aim to discern the efficacy of various platforms and systems relative to specific educational objectives and user needs. Establishing a repository of best practices and identifying the most impactful educational technologies will serve as a critical resource for educators and institutions. This guidance is fundamental for informed decision-making regarding the incorporation of VR and AR into medical curricula, ensuring that these tools are harnessed to their full potential for the enhancement of medical education.
5 Conclusions
This umbrella review systematically examined the use of VR and AR in medical education to determine their effectiveness in enhancing the competencies of healthcare professionals compared to traditional methods. Through a methodical application of PRISMA guidelines and the PICOS approach, 28 studies were evaluated using descriptive statistics and chi-square analysis to understand the impact of immersive technologies on educational outcomes. Our findings suggest that VR is the most researched.
Technology, with a significant portion of the studies reporting enhanced performance outcomes when VR is employed. The studies included in this review have shown a trend where VR and AR technologies are positively associated with improved learner engagement and performance.
However, the statistical analysis did not provide evidence of a significant association between the stages of medical education and the type of technology used. This indicates that while there is a positive trend towards the effectiveness of VR and AR in medical education, it is not yet statistically significant within the body of literature reviewed. It is important to note that the lack of significance may be attributable to the diverse methodologies, small sample sizes, and the heterogeneity of outcomes across the included studies.
The neutrality and non-evaluation of effects in some studies point to potential areas for further research. These gaps highlight the necessity for more rigorous, standardized, and larger-scale studies to conclusively determine the impact of VR and AR technologies in medical education. While immersive VR and AR technologies demonstrate promise in enhancing medical training, more comprehensive research is required to establish their definitive effectiveness. Future investigations should aim to overcome the limitations observed in this review, focusing on consistent reporting, larger sample sizes, and possibly meta-analytic approaches to provide a more robust assessment of these innovative educational tools.
Finally, a critical aspect to consider is the complexity and cost associated with VR technology. Despite a downward trend in prices, equipping each student with individual VR/AR systems for remote learning remains a complex and expensive endeavor. This reality points towards a potential model where future remote learning could occur in semi-centralized labs equipped with VR/AR technologies. In these settings, students could physically access the necessary equipment, while educators could engage and instruct remotely. This approach could offer a more feasible and cost-effective solution for integrating immersive technologies into medical education, especially in settings where individual ownership of such devices is impractical.
Data availability statement
The raw data supporting the conclusions of this article will be made available by the authors, without undue reservation.
Author contributions
TT: Conceptualization, Funding acquisition, Supervision, Writing – original draft, Writing – review & editing. DV: Investigation, Methodology, Writing – original draft, Writing – review & editing. PV: Investigation, Methodology, Writing – original draft, Writing – review & editing. LO: Investigation, Methodology, Writing – original draft, Writing – review & editing. CV: Conceptualization, Methodology, Supervision, Writing – original draft, Writing – review & editing.
The author(s) declare financial support was received for the research, authorship, and/or publication of this article.
This work has been supported by the Universidad Técnica Particular de Loja (grant No. POA_VIN-56).
Acknowledgments
The authors would like to thank Corporación Ecuatoriana para el Desarrollo de la Investigación y Academia—CEDIA for the support given to the present research, development, and innovation work through its CEPRA program, especially for the “Proyecto 18–Tecnologias Inmersivas” fund.
Conflict of interest
The authors declare that the research was conducted in the absence of any commercial or financial relationships that could be construed as a potential conflict of interest.
Publisher's note
All claims expressed in this article are solely those of the authors and do not necessarily represent those of their affiliated organizations, or those of the publisher, the editors and the reviewers. Any product that may be evaluated in this article, or claim that may be made by its manufacturer, is not guaranteed or endorsed by the publisher.
1. Kapoor S, Arora P, Kapoor V, Jayachandran M, Tiwari M. Haptics–touchfeedback technology widening the horizon of medicine. J Clin Diagn Res . (2014) 8(3):294–9. doi: 10.7860/JCDR/2014/7814.4191
PubMed Abstract | Crossref Full Text | Google Scholar
2. Herron J. Augmented reality in medical education and training. J Electron Resour Med Libr . (2016) 13:51–5. doi: 10.1080/15424065.2016.1175987
Crossref Full Text | Google Scholar
3. Rochlen L, Levine R, Tait A. First person point of view augmented reality for central line insertion training: a usability and feasibility study. Simul Healthc . (2017) 12:57. doi: 10.1097/SIH.0000000000000185
4. Nguyen M, Quevedo-Uribe A, Kapralos B, Jenkin M, Kanev K, Jaimes N. An experimental training support framework for eye fundus examination skill development. Comput Methods Biomech Biomed Eng Imaging Visual . (2019) 7:26–36. doi: 10.1080/21681163.2017.1376708
5. Childs B, Manganiello M, Korets R. Novel education and simulation tools in urologic training. Curr Urol Rep . (2019) 20:1–8. doi: 10.1007/s11934-019-0947-8
6. Walbron P, Common H, Thomazeau H, Sirveaux F. Evaluation of arthroscopic skills with a virtual reality simulator in first-year orthopaedic residents. Int Orthop . (2020) 44:821–7. doi: 10.1007/s00264-020-04520-1
7. Elzie C, Shaia J. A pilot study of the impact of virtually embodying a patient with a terminal illness. Med Sci Educ . (2021) 31:665–75. doi: 10.1007/s40670-021-01243-9
8. Gloy K, Weyhe P, Nerenz E, Kaluschke M, Uslar V, Zachmann G, et al. Immersive anatomy atlas: learning factual medical knowledge in a virtual reality environment. Anat Sci Educ . (2022) 15:360–8. doi: 10.1002/ase.2095
9. Lian W. Application of virtual reality technology and its impact on digital health in healthcare industry. J Commer Biotechnol . (2022) 27:152–60. doi: 10.5912/jcb1320
10. Yaqi M. Designing visual communications virtual reality matters in healthcare industry. J Commer Biotechnol . (2022) 27:120–6. doi: 10.5912/jcb1318
11. Falah J, Charissis V, Khan S, Chan W, Alfalah S, Harrison D. Development and evaluation of virtual reality medical training system for anatomy education. In Intelligent Systems in Science and Information 2014: Extended and Selected Results from the Science and Information Conference (2014) 591. p. 369–83. doi: 10.1007/978-3-319-14654-6_23
12. Ruthenbeck G, Reynolds K. Virtual reality for medical training: the state-of-the-art. J Simul . (2015) 9:16–26. doi: 10.1057/jos.2014.14
13. Lee D, Yi J, Hong J, Chai Y, Kim H, Kong H. Augmented reality to localize individual organ in surgical procedure. Healthc Inform Res . (2018) 24:394–401. doi: 10.4258/hir.2018.24.4.394
14. Alfalah S, Falah J, Alfalah T, Elfalah M, Muhaidat N, Falah O. A comparative study between a virtual reality heart anatomy system and traditional medical teaching modalities. Virtual Real . (2019) 23:229–34. doi: 10.1007/s10055-018-0359-y
15. Ivanov V, Klygach A, Shterenberg S, Strelkov S, Levy J. Advances in augmented reality (AR) for medical simulation and training. 3C Tecnol . (2020) 5:303–12. doi: 10.17993/3ctecno.2020.specialissue5.303-312
16. Alahmadi D, Bitar H, ALsaadi H, Boker L, Alghamdi L. Using augmented reality to enhance medical education in heart diseases: action design research. TEM J . (2021) 10:1141–8. doi: 10.3390/diagnostics10070500
17. Dhar P, Rocks T, Samarasinghe R, Stephenson G, Smith C. Augmented reality in medical education: students’ experiences and learning outcomes. Med Educ Online . (2021) 26:1953953. doi: 10.1080/10872981.2021.1953953
18. Khundam C, Sukkriang N, Noël F. No difference in learning outcomes and usability between using controllers and hand tracking during a virtual reality endotracheal intubation training for medical students in Thailand. J Educ Eval Health Prof . (2021) 18:1149220. doi: 10.3352/jeehp.2021.18.22
19. Albrecht T, Nikendei C, Praetorius M. Face, content, and construct validity of a virtual reality otoscopy simulator and applicability to medical training. Otolaryngol Head Neck Surg . (2022) 166:753–9. doi: 10.1177/01945998211032897
20. Prunoiu V, Popa D, Serbulea M, Brãtucu E, Simion L, Brãtucu M. Augmented reality in surgical oncology. A literature review. Chirurgia (Bucur) . (2022) 117:517–25. doi: 10.21614/chirurgia.2785
21. Toohey S, Wray A, Hunter J, Waldrop I, Saadat S, Boysen-Osborn M, et al. Comparing the psychological effects of manikin-based and augmented reality–based simulation training: within-subjects crossover study. JMIR Med Educ . (2022) 8:e36447. doi: 10.2196/36447
22. Lin H, Hwang G, Chou K, Tsai C. Fostering complex professional skills with interactive simulation technology: a virtual reality-based flipped learning approach. Br J Educ Technol . (2023) 54:622–41. doi: 10.1111/bjet.13268
23. McBain K, Chen L, Lee A, O'Brien J, Ventura N, Noël G. Evaluating the integration of body donor imaging into anatomical dissection using augmented reality. Anat Sci Educ . (2023) 16:71–86. doi: 10.1002/ase.2157
24. Amir-Behghadami M, Janati A. Population, intervention, comparison, outcomes and study (PICOS) design as a framework to formulate eligibility criteria in systematic reviews. Emerg Med J . (2020) 37(6):387. doi: 10.1136/emermed-2020-209567
25. Page M, McKenzie J, Bossuyt P, Boutron I, Hoffmann T, Mulrow C, et al. The PRISMA 2020 statement: an updated guideline for reporting systematic reviews. Int J Surg . (2021) 88:105906. doi: 10.1016/j.ijsu.2021.105906
26. Choi K, Chan S, Pang W. Virtual suturing simulation based on commodity physics engine for medical learning. J Med Syst . (2012) 36:1781–93. doi: 10.1007/s10916-010-9638-1
27. Gao K, Wiederhold B, Kong L, Wiederhold M. Methodology case study of the application of haptics to combat medic training programs. Ann Rev Cyberther Telemed . (2013) 191:53–7. doi: 10.3233/978-1-61499-282-0-53
28. Vankipuram A, Khanal P, Ashby A, Vankipuram M, Gupta A, DrummGurnee D, et al. Design and development of a virtual reality simulator for advanced cardiac life support training. IEEE J Biomed Health Inform . (2013) 18:1478–84. doi: 10.1109/JBHI.2013.2285102
29. Izard S, Juanes J, Palomera P. Virtual reality educational tool for human anatomy. J Med Syst . (2017) 41:1–6. doi: 10.1007/s10916-017-0723-6
30. Izard S, Juanes J, García F, Estella J, Ledesma M, Ruisoto P. Virtual reality as an educational and training tool for medicine. J Med Syst . (2018) 42:1–5. doi: 10.1007/s10916-018-0900-2
31. Yovanoff M, Chen H, Pepley D, Mirkin K, Han D, Moore J, et al. Investigating the effect of simulator functional fidelity and personalized feedback on central venous catheterization training. J Surg Educ . (2018) 75:1410–21. doi: 10.1016/j.jsurg.2018.02.018
32. Aksoy E, Izzetoglu K, Baysoy E, Agrali A, Kitapcioglu D, Onaral B. Performance monitoring via functional near infrared spectroscopy for virtual reality based basic life support training. Front Neurosci . (2019) 13:1336. doi: 10.3389/fnins.2019.01336
33. Drewett O, Hann G, Gillies M, Sher C, Delacroix S, Pan X, et al. A discussion of the use of virtual reality for training healthcare practitioners to recognize child protection issues. Front Public Health . (2019) 7:255. doi: 10.3389/fpubh.2019.00255
34. Hooper J, Tsiridis E, Feng J, Schwarzkopf R, Waren D, Long W, et al. Virtual reality simulation facilitates resident training in total hip arthroplasty: a randomized controlled trial. J Arthroplasty . (2019) 34:2278–83. doi: 10.1016/j.arth.2019.04.002
35. Starkov R, Zhang L, Bajka M, Tanner C, Goksel O. Ultrasound simulation with deformable and patient-specific scatterer maps. Int J Comput Assist Radiol Surg . (2019) 14:1589–99. doi: 10.1007/s11548-019-02054-5
36. De Ponti R, Marazzato J, Maresca A, Rovera F, Carcano G, Ferrario M. Pre-graduation medical training including virtual reality during COVID-19 pandemic: a report on students’ perception. BMC Med Educ . (2020) 20:1–7. doi: 10.1186/s12909-020-02245-8
37. Mariscal G, García E, Urías M, Duarte S, Pérez SM. Virtual reality simulation-based learning. Educ Knowledge Soc (EKS) . (2020) 21:11–6. doi: 10.14201/eks.20809
38. Mendes H, Costa C, da Silva N, Leite F, Esteves A, Lopes D. PIÑATA: pinpoint insertion of intravenous needles via augmented reality training assistance. Comput Med Imaging Graph . (2020) 82:101731. doi: 10.1016/j.compmedimag.2020.101731
39. Hood R, Maltby S, Keynes A, Kluge M, Nalivaiko E, Ryan A, et al. Development and pilot implementation of TACTICS VR: a virtual reality-based stroke management workflow training application and training framework. Front Neurol . (2021) 12:665808. doi: 10.3389/fneur.2021.665808
40. Liu P, Li C, Xiao C, Zhang Z, Ma J, Gao J, et al. A wearable augmented reality navigation system for surgical telementoring based on microsoft HoloLens. Ann Biomed Eng . (2021) 49:287–98. doi: 10.1007/s10439-020-02538-5
41. Mehrfard A, Fotouhi J, Forster T, Taylor G, Fer D, Nagle D, et al. On the effectiveness of virtual reality-based training for surgical robot setup. Comput Methods Biomech Biomed Eng Imaging Visual . (2020) 9:243–52. doi: 10.1080/21681163.2020.1835558
42. Qi D, Ryason A, Milef N, Alfred S, Abu-Nuwar M, Kappus M, et al. Virtual reality operating room with AI guidance: design and validation of a fire scenario. Surg Endosc . (2021) 35:779–86. doi: 10.1007/s00464-020-07447-1
43. Wolf J, Wolfer V, Halbe M, Maisano F, Lohmeyer Q, Meboldt M. Comparing the effectiveness of augmented reality-based and conventional instructions during single ECMO cannulation training. Int J Comput Assist Radiol Surg . (2021) 16:1171–80. doi: 10.1007/s11548-021-02408-y
44. Syed Abdul S, Upadhyay U, Salcedo D, Lin C. Virtual reality enhancing medical education and practice: brief communication. Digital Health . (2022) 8:20552076221143948. doi: 10.1177/2055207622114394
45. Plechatá A, Makransky G, Böhm R. Can extended reality in the metaverse revolutionise health communication? NPJ digital Medicine . (2022) 5:13. doi: 10.1038/s41746-022-00682-x
46. Andreasen E, Høigaard R, Berg H, Steinsbekk A, Haraldstad K. Usability evaluation of the preoperative ISBAR (identification, situation, background, assessment, and recommendation) desktop virtual reality application: qualitative observational study. JMIR Human Factors . (2022) 9:e40400. doi: 10.2196/40400
47. Cercenelli L, De Stefano A, Billi AM, Ruggeri A, Marcelli E, Marchetti C, et al. AEducaAR, anatomical education in augmented reality: a pilot experience of an innovative educational tool combining AR technology and 3D printing. Int J Environ Res Public Health . (2022) 19:1024. doi: 10.3390/ijerph19031024
48. Du W, Zhong X, Jia Y, Jiang R, Yang H, Ye Z, et al. A novel scenario-based, mixed-reality platform for training nontechnical skills of battlefield first aid: prospective interventional study. JMIR Serious Games . (2022) 10:e40727. doi: 10.2196/40727
49. Wang G, Badal A, Jia X, Maltz J, Mueller K, Myers K, et al. Development of metaverse for intelligent healthcare. Nat Mach Intell . (2022) 4:922–9. doi: 10.1038/s42256-022-00549-6
50. Heo S, Moon S, Kim M, Park M, Cha W, Son M. An augmented reality–based guide for mechanical ventilator setup: prospective randomized pilot trial. JMIR Serious Games . (2022) 10:e38433. doi: 10.2196/38433
51. Lombardo R, Walther N, Young S, Gorbatkin C, Sletten Z, Kang C, et al. Ready medic one: a feasibility study of a semi-autonomous virtual reality trauma simulator. Front Virtual Real . (2022) 2:719656. doi: 10.3389/frvir.2021.719656
52. Lu L, Wang H, Liu P, Liu R, Zhang J, Xie Y, et al. Applications of mixed reality technology in orthopedics surgery: a pilot study. Front Bioeng Biotechnol . (2022) 10:740507. doi: 10.3389/fbioe.2022.740507
53. Zikas P, Kateros S, Lydatakis N, Kentros M, Geronikolakis E, Kamarianakis M, et al. Virtual reality medical training for COVID-19 swab testing and proper handling of personal protective equipment: development and usability. Front Virtual Real . (2022) 2:6–11. doi: 10.3389/frvir.2021.740197
54. Barsom E, Graafland M, Schijven M. Systematic review on the effectiveness of augmented reality applications in medical training. Surg Endosc . (2016) 30:4174–83. doi: 10.1007/s00464-016-4800-6
55. Tatar İ, Huri E, Selcuk I, Moon Y, Paoluzzi A, Skolarikos A. Review of the effect of 3D medical printing and virtual reality on urology training withMedTRain3DModsim'Erasmus+ European union project. Turk J Med Sci . (2019) 49:1257–70. doi: 10.3906/sag-1905-73
56. Kyaw B, Saxena N, Posadzki P, Vseteckova J, Nikolaou C, George P, et al. Virtual reality for health professions education: systematic review and meta-analysis by the digital health education collaboration. J Med Internet Res . (2019) 21:e12959. doi: 10.2196/12959
57. Herur-Raman A, Almeida N, Greenleaf W, Williams D, Karshenas A, Sherman J. Next-generation simulation—integrating extended reality technology into medical education. Front Virtual Real . (2021) 2:115. doi: 10.3389/frvir.2021.693399
58. Viglialoro RM, Condino S, Turini G, Carbone M, Ferrari V, Gesi M. Augmented reality, mixed reality, and hybrid approach in healthcare simulation: a systematic review. Appl. Sci . (2021) 11:2338. doi: 10.3390/app11052338
59. Pallavicini F, Pepe A, Clerici M, Mantovani F. Virtual reality applications in medicine during the COVID-19 pandemic: systematic review. JMIR Serious Games . (2022) 10:e35000. doi: 10.2196/35000
60. Vayssiere P, Constanthin P, Herbelin B, Blanke O, Schaller K, Bijlenga P. Application of virtual reality in neurosurgery: patient missing. A systematic review. J Clin Neurosci . (2022) 95:55–62. doi: 10.1016/j.jocn.2021.11.031
61. Clarke E. Virtual reality simulation—the future of orthopaedic training? A systematic review and narrative analysis. Adv Simul . (2021) 6:1–11. doi: 10.1186/s41077-020-00153-x
62. Bansal G, Rajgopal K, Chamola V, Xiong Z, Niyato D. Healthcare in metaverse: a survey on current metaverse applications in healthcare. Ieee Access . (2022) 10:119914–46. doi: 10.1109/ACCESS.2022.3219845
Keywords: immersive technologies, virtual reality, augmented reality, medical education, medical training and learning
Citation: Tene T, Vique López DF, Valverde Aguirre PE, Orna Puente LM and Vacacela Gomez C (2024) Virtual reality and augmented reality in medical education: an umbrella review. Front. Digit. Health 6:1365345. doi: 10.3389/fdgth.2024.1365345
Received: 4 January 2024; Accepted: 4 March 2024; Published: 14 March 2024.
Reviewed by:
© 2024 Tene, Vique López, Valverde Aguirre, Orna Puente and Vacacela Gomez. This is an open-access article distributed under the terms of the Creative Commons Attribution License (CC BY) . The use, distribution or reproduction in other forums is permitted, provided the original author(s) and the copyright owner(s) are credited and that the original publication in this journal is cited, in accordance with accepted academic practice. No use, distribution or reproduction is permitted which does not comply with these terms.
*Correspondence: Talia Tene [email protected] Cristian Vacacela Gomez [email protected]
Disclaimer: All claims expressed in this article are solely those of the authors and do not necessarily represent those of their affiliated organizations, or those of the publisher, the editors and the reviewers. Any product that may be evaluated in this article or claim that may be made by its manufacturer is not guaranteed or endorsed by the publisher.

An official website of the United States government
The .gov means it’s official. Federal government websites often end in .gov or .mil. Before sharing sensitive information, make sure you’re on a federal government site.
The site is secure. The https:// ensures that you are connecting to the official website and that any information you provide is encrypted and transmitted securely.
- Publications
- Account settings
Preview improvements coming to the PMC website in October 2024. Learn More or Try it out now .
- Advanced Search
- Journal List
- J Adv Med Educ Prof
- v.6(3); 2018 Jul
The Applications of Virtual Reality Technology in Medical Groups Teaching
Mahnaz samadbeik.
1 Department of Health Information Technology, Social Determinants of Health Research Center, Lorestan University of Medical Sciences, Khorramabad, Iran
DONYA YAAGHOBI
Peivand bastani.
2 Health Human Resources Research Center, School of Management and Medical Informatics, Shiraz University of Medical Sciences, Shiraz, Iran
SHAHABEDDIN ABHARI
3 School of Allied Medical Sciences, Tehran University of Medical Sciences, Tehran, Iran
RITA REZAEE
4 Clinical Education Research Center, Shiraz University of Medical Sciences, Shiraz, Iran
ALI GARAVAND
5 School of Allied Medical Sciences, Shahid Beheshti University of Medical Sciences, Tehran, Iran
Introduction:
Virtual reality is a new method for training different medical groups. Based on this technology, professionals and students of various medical sciences can determine their level of competence for medical treatment before any performance on the patient. Therefore, the aim of this study was to identify the applications of virtual reality technology for training the medical groups.
This is a scoping review study conducted in 2016. Articles were retrieved through the search of related keywords in databases such as Pub Med, Scopus, Web of Sciences, Springer, and Google scholar. Then, after applying the entry criteria, 21 papers were selected from a total of 1343. Data extraction was done by a data collection form. The collected data were summarized and reported using content analysis technique according to the study purpose.
The findings of the study indicated that 11 cases (48%) have used virtual education technology for laparoscopic surgery training. Using virtual reality has improved learning in 17 (74%) studies. A higher accuracy in medical practice by people trained through VR has been reported in 20 (87%) studies.
Conclusion:
The results indicate that the application of virtual reality capabilities plays an important role in improving the performance of different medical groups. According to the results, it can be suggested that virtual reality capabilities should be used to train different medical groups based on their individual and collective needs.
Introduction
Virtual reality is a new technology that has been widely used in the health field in recent years and it is applied in a wide range of diseases. In fact, this technology is the simulation of the peripheral world through a computer as well as communication through a receiver ( 1 ). Virtual reality consists of output tools (vision, hearing, tactile and power transmitter), input devices (mouse, chaser, gloves, etc.), a virtual environment’s graphical manufacturing system as well as an information software. In a virtual environment, all the features of activity such as duration, severity and type of feedback can be adopted based on the type of treatment and individuals’ ability ( 2 , 3 ). In addition, individuals can see their motor results and correct them if necessary.
One of the most important applications of VR in medical science is in medical education, which has been widely used in the last decade. Many studies have paid attention to various VR applications in medical education ( 4 , 5 ), including the understanding of fracture anatomy by orthopedic residences ( 6 ), improving the knowledge and skills of clinical residences in the MICU and as an adjunct complement for standard clinical education if internal residences in MICU, as well as general objections regarding the patient safety, ethical concerns and financial constraints for education of medical students have led to a widespread application of non-human and inanimate models for training the laparoscopic skills because simulation-oriented syllabus is very effective in transferring skills to the operating room ( 7 - 13 ).
The American Board of Internal Medicine (ABIM) has announced that it is better for residents to be trained by simulation tools before attempting any interventions on patients because it has been effective in performing invasive hemodynamic monitoring, mechanical ventilation, and standardized educational intervention ( 14 ).
Stefanidis et al. concluded that suture training using simulator improves the speed and mobility of practitioners in the operation room ( 15 ). In the same line, Lin et al. found that given the fact that performing bone cut or bone surgery requires high experience and sensitivity, training the simulation of virtual and tactical surgery with feedback can be a safe, repeatable and cost-effective method compared to traditional methods ( 16 ).
Given the importance of medical education using the virtual reality tools and its role in improving the quality of medical education in various medical specialties, many papers examined this field in recent years. Although Walsh et al. instead of using conventional methods systematically reviewed education of endoscopy using virtual reality, but there was no systematic study regarding its applications ( 17 ). Therefore, the present study was a systematic review that aimed at identifying the applications of virtual reality in training various medical groups.
This study is a scoping review which aimed to identify virtual reality applications in training the medical groups in 2016. The research question was identified based on the PCC (Population, Concept and Context) elements. This particular question includes: medical groups that use VR for training (Population); the effect of Virtual Reality in Medicine Groups training (Concept); use of Virtual Reality in medical teaching centers (context).
Consistent with Best Evidence Medical Education (BEME) recommendations, the search was performed on valid databases as well as PubMed, Scopus, ISI Web of Science and Springer. During the search, the restrictions and related keywords were retrieved. To search for related papers, the combination of keywords ( Table 1 ), with the English language and 5-year period restrictions were applied. Also, a search expert helped us to improve the quality of searches.
The search strategy of the research
The inclusion criteria were clinical trial and exclusion criteria were commentary non-randomized trials and non-clinical trials. Erratum Review, Commentary, contact with Editor and available Studies were considered. The process of selection of articles was based on the PRISMA chart ( Figure 1 ), and 24 articles were selected out of 1343 retrieved articles. In accordance with BEME, all stages of the selection and evaluation of the quality of the articles were done by two researchers and in the case of disagreement, a third person was available to help.

The PRISMA flowchart for the paper selection process
Considering BEME, to assess the quality of the studies, we excluded Consortium Assessment Tool 2010 (was used) and 3 papers with undesirable quality from the study. Finally, 21 qualified papers of clinical trials were selected.
Consistent with BEME, the required information of the selected papers was collected using a data collection form (including items of the type of study, the year of the study, the sample number, the country where the study was conducted, first author, the most important findings, the proposed solutions and the limitations of each study) and the data were summarized and reported in Tables according to the objectives of the study.
All of 21 clinical trials with inclusion criteria were selected for the current study. 2013 had the largest number of studies ( 9 ). Studies were conducted in Denmark (4 studies), Canada, the United States and Germany (3 studies for each), England and Australia (2 studies for each), and the Netherlands, Switzerland, Norway, and Austria, (each with 1 study). Using pre-test and post-test was the most common method for data collection.
Based on the other results, the effect of using virtual reality was ranked from 1-4 (very effective, effective, ineffective, and negative); only two studies reported the negative effect on the use of virtual reality in training medical groups. In addition, two studies have reported the ineffectiveness of the use of virtual reality in medical education.
The findings of the present study indicated that the average period of training different medical groups using virtual reality was between six months to one year. In addition, according to the findings of the present study, the application of virtual reality reduced the time required for training medical groups. The secondary results of this study are shown in Table 2 to address the advantages and disadvantages of virtual reality in teaching medical groups.
Advantages and disadvantages of training various medical groups using virtual reality
Other secondary results of the current study include suggestions for improving the application of virtual reality that are presented in Table 3 .
Proposals for the improvement of the application of virtual reality for training various medical groups
The results indicated that all studies were conducted in developed countries. 95% of the studies that have been studied emphasize the improvement of the skills of trainees (various medical groups) using virtual reality although a few studies noted the ineffectiveness of virtual reality on medical education. Julie Enne et al. proposed the implementation of virtual reality training courses for the acquisition of hysteroscopic skills for clinical professionals who are interested in learning sterilization techniques ( 36 ).
The application of virtual reality in training different medical groups includes different effects. That is, in some specialties virtual reality is very effective, and in several studies and specialties no positive effects are noted regarding the use of virtual reality compared to the control group. A recent study on gynecology and obstetrics reported the impact of VR application on the enhancement of infant and maternal health ( 35 ).
A study conducted in 2012 concluded that simulation-based education significantly increased the skills and knowledge of residents in the intensive care unit. It is an invaluable approach for standard medical education ( 28 ). However, Brydges et al. indicated that "contrary to expectations of researchers, intervention group did not have more advantages over self-regulated learning (SRL), and in fact they spent more time on doing it" ( 20 ). However, most studies reported the positive impact of the application of virtual reality for training the medical groups. Given that a small number of studies reported the lack of effectiveness of the virtual reality application, further studies are required to be conducted in this area.
In addition, Bongers et al. noted that "this study proposed that multitasking could be taught using virtual reality simulator equipment. But the effectiveness of surgery reduced in trainees with multiple trainings and this level of skill requires more research" ( 18 ).
The results emphasize a better understanding of the exterior and interior spatial relationships of the organs and development of the anatomical knowledge of students through the application of VR. Yanping et al. emphasized the improvement of the skills of beginners and experienced bone sawing rescuers because the application of surgical simulations helps the trainees to increase their recognition skill of the anatomical positions ( 16 ). Therefore, virtual reality can be used for training the anatomy courses of different medical groups.
Other results showed that virtual reality should be used as a complementary training along with main training and it can never replace the training in the real environment. A study in the field of software development of virtual reality used for education of nursing emphasized guidance in the objectives of simulation training software and its combination with conventional trainings ( 40 ). In addition, some studies pointed the effective and positive effects of simulation-oriented syllabus on transferring skills to the operating rooms ( 8 - 13 ).
The results revealed that the presence of an instructor is very influential (guided-training) since his absence may lead to disruption in the learning process and the performance can be reduced ( 22 , 26 ).
One of the issues of conducting this area is the high cost of these studies, such as the high cost of monitors, programming, implementation environment, participants, and so on. A higher number of student were proposed in a study to cover the high cost of using virtual reality ( 39 ).
Given the importance of continuous monitoring of education, the use of feedback during training is recommended ( 17 , 26 , 32 , 37 , 38 ). Also, the use of virtual reality is suggested for holding retraining courses to increase the quality of the educations.
The results showed that people trained by virtual reality had lower performance errors and higher accuracy compared to those trained by conventional approaches. For example, many studies in the area of education using the simulator indicated that inexperienced residents who learned colonoscopy and endoscopy in the simulator before doing them in real-case (on the patient and in the operating room) did much better and with less errors than other residents who learned it directly in the real environment ( 41 - 44 ).
The application of virtual reality, as a complementary method, plays an important role in improving the performance of different medical groups. Therefore, given the results, it is suggested that it should be used as a complementary educational tool along with main education. Considering that laparoscopic surgery is one of the most important surgical procedures that is taught using virtual reality, individual and general factors affecting its exact implementation should be identified. In addition, given the importance of the issue, it is suggested that more studies should be carried out on the training of medical groups using virtual reality to determine the type and extent of its impact on the performance and efficiency of the students. With respect to the results of the present study, it is suggested that virtual reality should be used in training such skills as laparoscopic surgery, education of orthopedic residents, Gynecology residents, suturing, ultrasound, nursing procedures and paramedical interventions. In addition, it seems necessary to do studies to determine the effectiveness and usefulness of the method and satisfaction of the trainees and patients. Furthermore, considering the needs of trainees, designing virtual reality tools can be an appropriate solution for its effective use for training the medical groups.
Acknowledgement
This study is the result of a research project done through the financial support of Health Human Resources Research Center, School of Management and Medical Informatics, Shiraz University of Medical Sciences with grant No. 95-01-68-12720.
Conflict of Interests: None declared.
Virtual Reality in Health Care
Ieee account.
- Change Username/Password
- Update Address
Purchase Details
- Payment Options
- Order History
- View Purchased Documents
Profile Information
- Communications Preferences
- Profession and Education
- Technical Interests
- US & Canada: +1 800 678 4333
- Worldwide: +1 732 981 0060
- Contact & Support
- About IEEE Xplore
- Accessibility
- Terms of Use
- Nondiscrimination Policy
- Privacy & Opting Out of Cookies
A not-for-profit organization, IEEE is the world's largest technical professional organization dedicated to advancing technology for the benefit of humanity. © Copyright 2024 IEEE - All rights reserved. Use of this web site signifies your agreement to the terms and conditions.
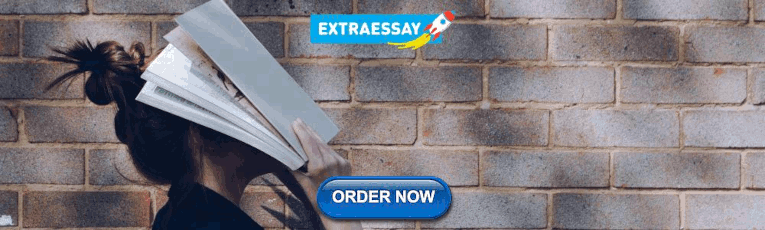
IMAGES
VIDEO
COMMENTS
Principal Findings. This bibliometric analysis of 8399 publications on VR research in medicine revealed that the field began to develop in the 1990s, grew in the 2000s, and has been thriving in the 2010s in terms of both publications and citation counts. Original articles accounted for 63.1% of the literature.
and research experts in the field (n = 3) ... D Gromala, G Tao, E Cordingley, C Sun. Virtual reality clinical research: promises and challenges, JMIR Serious Games 2018;6(4). 10.2196/10839. JMIR ... et al. Child and parent perspectives on the acceptability of virtual reality to mitigate medical trauma in an infusion center. Matern ...
The healthcare industry has become a big adopter of Virtual Reality (VR) technology. This paper demonstrates how healthcare and medical professionals can use VR technology for surgery and personal ...
Screen-based learning. Confusingly, screen-based learning has previously been referred to as 'virtual reality' in the medical literature. However, there is now an understanding that the value of virtual reality comes from immersion and the sense of presence - the feeling of 'being there' - that it generates. 3,4 As such, only VR that is immersive - using headsets that completely ...
Several 3D virtual reality (VR) modules are in use in the field of cardiac interventions. The applications include skills simulation and training, periprocedural guidance and planning, enhanced 3D ...
Abstract Virtual reality (VR) and augmented reality (AR) are aspiring, new technologies with increasing use in critical care medicine. While VR fully immerses the user into a virtual three-dimensional space, AR adds overlaid virtual elements into a real-world environment. VR and AR offer great potential to improve critical care medicine for patients, relatives and health care providers. VR may ...
Pulse!! A Model for Research and Development of Virtual-Reality Learning in Military Medical Education and Training. 36: Dyre et al. (2016) Collecting Validity Evidence for the Assessment of Mastery Learning in Simulation-Based Ultrasound Training. 37: Ende et al. (2012) Strategies for training in diagnostic upper endoscopy: a prospective ...
Virtual reality applications in medical field The applications of this technology are in psychological therapy, medical rehabilitation, medical research and teaching; thus, students can now perform safe and controlled surgery virtually without any risk. 20 , 29 It is helpful to learn a life-saving skill during accidental and emergency cases.
Immersive VR refers to a simulated virtual environment delivered to a user via visual, auditory, and sometimes haptic stimuli through a head-mounted display (HMD). 1 The immersive aspect is derived from interactivity and tracking of the user's head movements, creating spatial presence within the virtual world. 2 Augmented reality refers to the integration and superimposition of digital ...
Virtual reality (VR) and augmented reality (AR) are emerging technologies that allow various applications, ranging from immersive entertainment or educational experiences to medical care. VR is defined as the user's complete immersion into a virtual three-dimensional environment, while AR retains the connection to the real world but ...
This paper studies publication trends of VR-related medical and health research in general and in particular subfields (e.g. VR in surgery). The study of such publication patterns has indicated emerging technology trends and uptakes in different medical fields [13,14,15].Such information is especially interesting in the field of VR where the lack of systematic analysis still hampers the wide ...
Mixed Reality (MR) is an emerging technology that represents the converg ence of both Virtual Reality. (VR) and Augmented Reality (AR) into a seamless and integrated experience. MR combines ...
In recent years, the development of virtual reality (VR) has progressed significantly. 1 VR, due to its ability to design a standard, reproducible, and controllable environment, 2 has been increasingly used from simple graphical application to advanced usages in the automobile industry, architecture, and medicine 3 as well as the production, the visualization of science, engineering, and ...
Methods. After a brief description of VR technology, we discuss the past 20 years of clinical VR applications in the medical field. Then, we discuss the theoretical basis of the combination of VR technology and CAM, the research thus far, and practical factors regarding usability, etc., from the following three main aspects: clinical application, teaching, and scientific research.
Advanced visualization of medical imaging has been a motive for research due to its value for disease analysis, surgical planning, and academical training. More recently, attention has been turning toward mixed reality as a means to deliver more interactive and realistic medical experiences. However, there are still many limitations to the use of virtual reality for specific scenarios. Our ...
Virtual reality (VR) technology has the potential to revolutionize the way medical professionals diagnose and treat patients. The use of VR in the medical field is a rapidly growing area of research, with potential applications ranging from surgical training and rehabilitation to pain management and psychological therapy. However, several barriers still need to be overcome for VR to be widely ...
View PDF. Virtual reality (VR) is a potentially powerful technology for enhancing assessment in mental health. At any time or place, individuals can be transported into immersive and interactive virtual worlds that are fully controlled by the researcher or clinician. This capability is central to recent interest in how VR might be harnessed in ...
There have been many major developments in the use of augmented reality (AR), virtual reality (VR), and mixed reality (MR) technologies in the context of global surgical research, yet few reports on the trends in this field have been published to date. This study was therefore designed to explore these worldwide trends in this clinically important field. Relevant studies published from 1 ...
Virtual Reality (VR) is a developing technology, which seems to have extensive applications in different areas such as entertainment, sports, gaming and simulation. In the current scenario, due to Computer-generated imagery and content aim at simulating a real presence through senses capability, it has functional applications in the medical field. So, there are requirements to study its ...
11. Falah J, Charissis V, Khan S, Chan W, Alfalah S, Harrison D. Development and evaluation of virtual reality medical training system for anatomy education. In Intelligent Systems in Science and Information 2014: Extended and Selected Results from the Science and Information Conference (2014) 591. p. 369-83. doi: 10.1007/978-3-319-14654-6_23
Results: The findings of the study indicated that 11 cases (48%) have used virtual education technology for laparoscopic surgery training. Using virtual reality has improved learning in 17 (74%) studies. A higher accuracy in medical practice by people trained through VR has been reported in 20 (87%) studies.
Virtual reality (VR) and augmented reality (AR) technology hold potential across many disciplines in medicine to expand the delivery of education and healthcare. ... VR-AR has already found diverse applications within the medical field. ... Silva E, Breslau J, Barr RM, et al. ACR white paper on teleradiology practice: a report from the Task ...
This paper presents a prime aspect of Augmented and Virtual Reality development in the field of healthcare. We explored several recent works and articles and a comparison between generic application development and immersive technology-based application is included. The paper talks about more practical approaches that can be taken to enhance the effectiveness of the application. The resources ...