Academia.edu no longer supports Internet Explorer.
To browse Academia.edu and the wider internet faster and more securely, please take a few seconds to upgrade your browser .
Enter the email address you signed up with and we'll email you a reset link.
- We're Hiring!
- Help Center
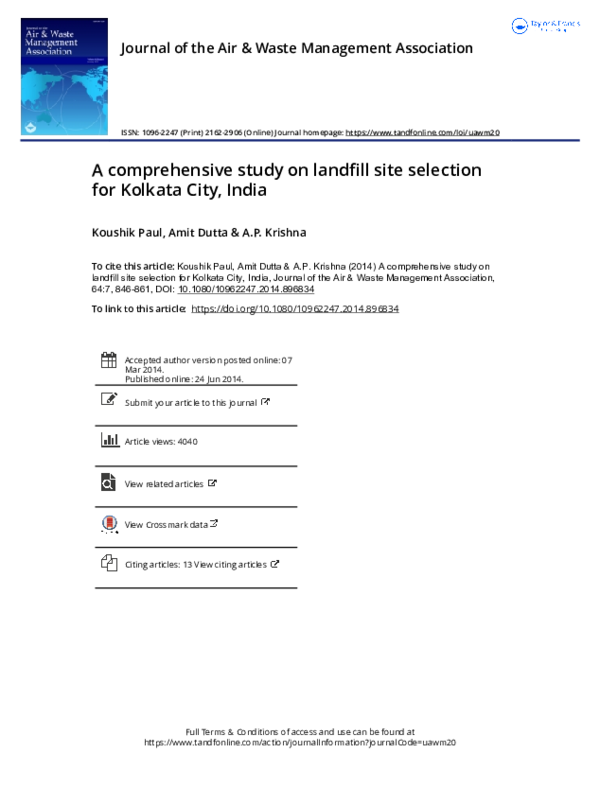
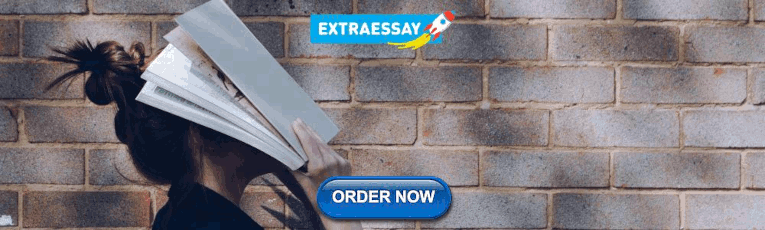
A comprehensive study on landfill site selection for Kolkata City, India

2014, Journal of the Air & Waste Management Association
Related Papers
International Journal of Research -GRANTHAALAYAH
Amit Prakash multaniya , Sanju Verma
Currently, the solid waste management work of Raipur is being done in a completely traditional way, which is very expensive and time consuming. The site selection method using Geospatial techniques can function as a decision support tool for enhanced efficient and effective management of municipal solid waste. This Study's aim was to develop landfill siting by crossing major barriers such as political, economic and environmental pressures for the execution of a suitable site criteria by employing combination of geospatial technique and Weighted Linear Combination (WLC) of Multi-Criteria Decision Analysis (MCDA) in Raipur urban area. The preliminary step of the methodology was geospatial operation and Study that disqualified all areas unsuitable for landfill siting. The insularity of suitable criteria for landfill site was generated based on published information on solid waste management, existing laws and regulatory necessities, as well as the existing local conditions. The different suitability criteria considered are soil, land use land cover, digital elevation model (DEM), surface waters, transport network, lithology and geological structures. Criteria were mapped using the geospatial technique; each criterion was identified and weighted by score for overlay to create suitability maps using GIS. The final results showed that there are more locations for landfills for Raipur urban area. In the present Study there are sporadic areas identified as suitable sites for landfill location. In the site suitability analysis 74.79% area found not suitable for landfill siting, 20.93% least suitable and 3.25% moderate suitable. Out of the remaining area, 1.03% area is found most suitable.
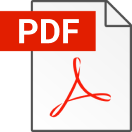
Bikash Sherchan
Solid waste management (SWM) is a significant aspect of infrastructure development and is linked in the direction of longterm sustainability and aids in reducing waste's social and economic repercussions of waste on the environment. Furthermore, the requirement is felt more acutely in developing nations as a result of increased trash generation as a result of poor waste management systems combined with rapid population expansion. Landfill sites are an inevitable element of a city's waste management; proper site selection is critical and has a significant impact on the city's physical, environmental, and socioeconomic aspects. Pre-existing conditions of Nepalese restructuring and capacity constraints in newly established municipalities have not been able to give sufficient answers to landfill site siting concerns in these towns. As a result, for complex decision-making, a reasonable strategy is required, necessitating input from diverse stakeholders and organizations in c...
Rohit Gowda
Solid waste management is one of the major problems being faced by the developing countries. The sensitivity of each parameter towards dumping of municipal solid waste in the landfilling site has to be analyzed carefully. Otherwise, it may alter the ecological and environmental balance of the surrounding area. In this study, the identification of a potential landfill site for Mysore city was done in 3 stages. In the first stage, 14 wastelands were identified and considered as potential landfilling sites. In the 2nd stage, suitability index and weightages for selective parameters were defined with the help of extensive literature survey. In the final stage, the sensitivity of the selected wastelands for various environmental and socio-economical parameters was analyzed using weighted sensitivity method. The result obtained was then utilized for decision making purpose for selecting an optimum landfill site.
Proceedings of the 6th International Conference on Solid Waste Management (6th IconSWM 2016) “Waste Management & Resource Utilisation”. (Ed: Prof. Sadhan Kumar Ghosh)
Koushik Paul , Amit Dutta
Kolkata is one of the four major metropolitan cities in India and capital city of the state of West Bengal. With an area of 187.33 km2 and a population of about 10 million (including a floating population of about 6 million), the city generates about 3500 MT of solid waste daily. Currently Kolkata Municipal Corporation (KMC) disposes its waste at Dhapa (21.47 ha), where the disposal rate exceeds 3000 MT/day, and at Garden Reach (3.52 ha) where the disposal rate is around 100 MT/day. Considering the exhaustion of Dhapa landfill space, city planners are urgently searching for an alternate disposal ground. National Environmental Engineering Research Institute (NEERI), under the sponsorship of Central Pollution Control Board (CPCB) has documented the site selection criteria for municipal solid waste disposal ground to suit Indian conditions. The developed criteria accounts for environmental conditions, accessibility, geological and hydrogeological conditions, ecological and societal effects. The paper attempts to locate the most suitable site for disposal of KMC-area solid waste using the multicriteria decision analysis as stipulated in CPCB 2003 guidelines and the overlay analysis of Geographic Information System (GIS).
The haphazard growth in the urban population of the Indian cities in the recent past is creating a great demand for solid waste management. In developing countries solid waste management is becoming a major public health and environment concern. This trend can be linked to our changing food habits, lifestyles and living standard. Due to less priority, limited funds and poor planning many Indian cities dump their solid waste in open places. Aurangabad with a population of around 1.2 million is one of the major city in Maharashtra which currently generates around 400 tons of solid waste. Currently Aurangabad Municipal Corporation (AMC) disposes its waste at exhausted landfill at Naregaon (8.9 ha) which is about 5km from city. Considering the exhaust condition on Naregaon landfill, municipality planners are searching for another land in the vicinity but in vain due to severe oppose from local people. Motivated by Aurangabad Municipal Corporation in developing the city, this paper tries to find the suitable landfill sites using multi criteria decision analysis, overlay analysis of Geographic Information System (GIS) and Site Suitability.
Arabian Journal of Geosciences
roopjyoti hazarika
Kumar Devendra
KN - Journal of Cartography and Geographic Information
ateeque ahmad
The government authorities in developing countries are awfully concerned with coping out the problems of the rising issues related to the disposal of solid wastes. Most of the Indian cities still dispose of solid waste unscientifically causing to severe environmental as well as public health problems. Geographic information systems (GIS) and analytic hierarchy process (AHP) have emerged as efficient tools for multiple criteria decision analysis (MCDA) in effective solutions of solid waste management. The present study focuses on the integration of GIS and AHP in identifying potential sanitary landfill areas for solid waste disposal in Durgapur city, West Bengal, India. Eleven criteria were selected viz. land elevation, slope, soil, geology, land use land cover, distance to surface water, distance to tube wells, distance to roads, distance to industrial belts, distance to sensitive places, and land cost. All the criteria were aggregated using weighted overlay analysis in GIS environm...
editor of J E T I R Research journal
Selection of Solid Waste Landfills for Developing Townships in India: A GIS Based Case Study
IRJES JOURNAL
Open dumping is the most common method of solid waste disposal in many developing countries including urban areas. Appropriate landfill site selection is important to minimize negative impacts associated with open dump sites. Landfill siting is an extremely difficult task to accomplish due to strong public opposition and regulations. Developing countries do not have a systematic process for landfill site selection and hence unsuccessful landfill sitting leading to environmental degradation is typically the result especially in the developing world. Selection criteria include engineering, environmental and economic criteria. Geographical Information System(GIS) is a framework for storage, maintenance, management and analysis of geographical data and it has beendesigned for working with data that has spatial and descriptive dependency.No site selection study focusingon waste disposal has been performed in Bareilly town of Uttar Pradesh, India , which is located at 250 Km East of Delhiand have a population of over 9.5 lakh people and total waste production ofapprox.129813Metric tons per year. This study hasbeen done using Guidelines for Selection of site on different factors in which criteria such as distance from residential areas,distance from roads, land use, distance from ground water, distance from faults, geology, distance from sensitiveecosystems, etc. were used and after data geo referencing, the weighting of the criteria and adjusting them with the geographical features of the area, data overlaid and finally three locations proposed for landfill were introduced in Bareilly town. Among the proposed areas, one was selected as the best location according to thehypotheses. The obtained results of this study may be helpful for policy makers of Bareilly town.Data were collected from Bareilly district, India
RELATED PAPERS
De Uil van Minerva
Walter Van Herck
Proceedings of the Latvian Academy of Sciences. Section B. Natural, Exact, and Applied Sciences.
Modra Murovska
Onomázein Revista de lingüística filología y traducción
Joaquín Martínez Sánchez
E-fabulations: e-journal of children's …
Tomas Toscano
E-Review. Rivista degli Istituti Storici dell'Emilia-Romagna in Rete
gianluca gabrielli
IOP Conference Series: Materials Science and Engineering
Tamás Mohay
Iuliana Toma-Dasu
Letters in Applied Microbiology
ESTER SUNEN
Revista Brasileira de Políticas Públicas
Pedro Nicoli
IEEE Transactions on Components, Packaging and Manufacturing Technology
ARAFAT KABIR 1910030
Mourad Baiou
The American journal of tropical medicine and hygiene
Ahmad Rufa'i Abdullahi
Érica Siqueira
Brazilian Journal of Infectious Diseases
Maria Madel Aquino
Jamal Harimudin
The Journals of Gerontology Series A: Biological Sciences and Medical Sciences
Carla Caba Flores
Johana Alexandra Pulido Castelblanco
2016 16th Mediterranean Microwave Symposium (MMS)
Mihai Sanduleanu
Eleuterio R Ruiz
Revista do Hospital das Clínicas
anderson carvalho
François Odinet
Tibebu Yohannis
Nadezhda Berezina
See More Documents Like This
RELATED TOPICS
- We're Hiring!
- Help Center
- Find new research papers in:
- Health Sciences
- Earth Sciences
- Cognitive Science
- Mathematics
- Computer Science
- Academia ©2024
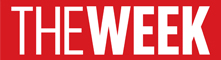
- THE WEEK TV
- ENTERTAINMENT
- WEB STORIES
- JOBS & CAREER
- Home Home -->
- News News -->
- Business Business -->

India’s answer to the mounting landfill problem: Bio-mining and Bio-remediation
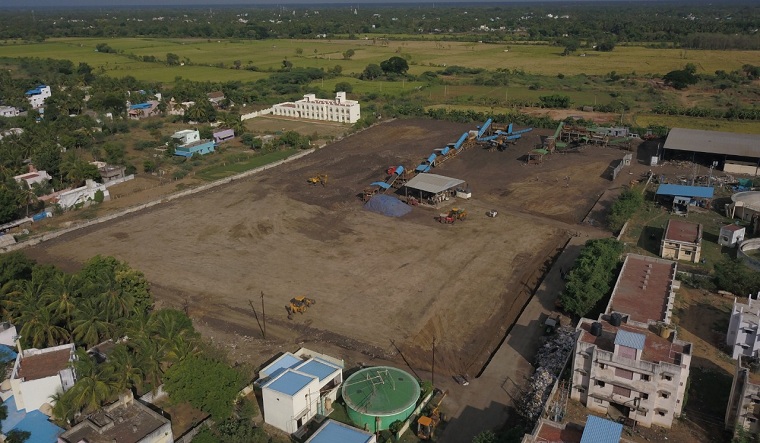
The smelly garbage mountains in our cities—with hawks hovering over them and stray dogs, cows and rats wandering at will—are the greatest reminders of our irresponsible attitude. Years of neglect and indiscriminate dumping of Municipal Solid Waste (MSW), generated from households and bulk waste generators like apartment complexes and hotels, formed these massive mountains. Many countries practise open dumping as a final disposal method for MSW, which not only causes surface water pollution, but the leachate (dark liquid) discharge from the dumpsite also pollutes the groundwater irreversibly. A dumpsite containing 0.1 million tonne of waste reportedly has a potential to generate 2,770 tonne of methane every year, which is equivalent to 69,250 tonne of carbon dioxide emissions. Methane is estimated to have a global warming potential that is 84 times greater than carbon dioxide. To make this worse, methane in the landfills is particularly dangerous―t often auto-ignites, causing fires in dumpsites and generating smoke and emissions, thereby causing severe air pollution.
What is bio-mining?
Governments around the world have recognised the problem and over the years landfill mining or bio-mining has become a popular method of excavating waste from active or closed landfills and segregating the aggregates generated with an ultimate purpose to clear them and reclaim the land. Urban India accounts for a third of India’s population and generates 54.75 million tonne of MSW annually. From 2014 onwards, the Swachh Bharat Mission has been emphasising on reclamation of landfill sites to adhere to the Solid Waste Management (SWM) Rules, 2016, and the guidelines of the Hon National Green Tribunal, with an aim to recover over an estimated 10,000 hectares of urban land that is locked in these dumpsites in India. Urban India has been struggling to set up infrastructure in solid waste management, which requires a huge land bank, and land reclamation could truly solve this problem and enable a turnaround in scientific management of MSW in the country.
Bio-mining was mandated by the SWM Rules, 2016, wherein it was a preferred methodology over ‘capping’ which simply meant covering the waste with soil. This, however, did not solve the problem of water pollution that continued unabated and the land could not be reclaimed.
B Dharmaraj, Managing Director, Zigma Global Environ Solutions Private Limited, which offers Landfill Mining services, says, “Landfills are a continuous source of pollution, and the only solution is to mine and process them. Until this happens, the water, air and soil contamination will continue steadily. Integrated landfill mining enables complete outsourcing of activity by the urban and local bodies (ULBs), thus enabling a dedicated approach toward reclamation.”
This is where bio-mining or landfill mining becomes a viable solution for the reclamation of these large sites. Different fractions of aggregates are segregated from the dumpsite and recycled, reused or repurposed in a suitable way, thereby cleaning the environment and also resulting in financial value and resource flow. Bio-mining is the best way to remediate open dumpsites to achieve zero emission of landfill gases and leachate, and reclamation of land to be used for other purposes.
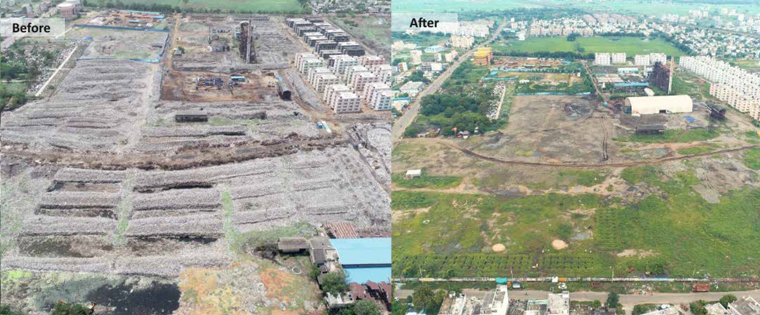
“There is a misconception that bio-mining is just segregation,” says A. Rajasekaran, President- Technical, Zigma Global Environ Solutions Pvt. Ltd. “These landfills are quite often biologically active, owing to the haphazard dumping, which does not allow the organics to degrade completely. Hence, the waste needs to be first stabilised or bio-remediated, as a result of which the fly menace, odour, scavenging animals and birds just vanish. It also results in the moisture getting onto optimum levels wherein the waste is now apt for segregation and processing.”
Challenges of bio-mining
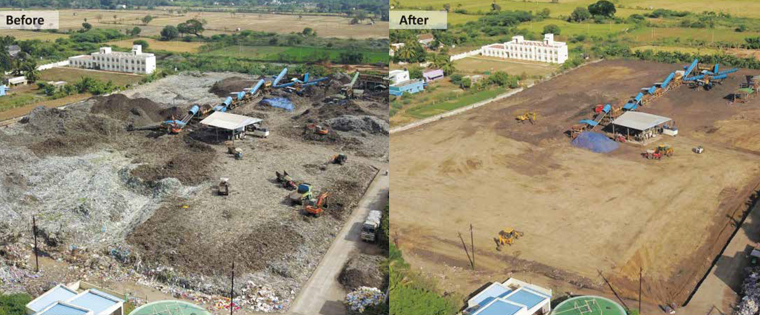
The CPCB Guidelines on Disposal of Legacy Waste 2019 provides a detailed methodology on how the process has to be carried out and the means by which the aggregates generated can be responsibly disposed. This requires sophisticated machinery and a flawless approach towards maintaining the quality of aggregates so that they can be disposed responsibly. In India, the waste is delivered in the landfills after multiple levels of scavenging, as a result of which most of the aggregates which can fetch commercial value cease to exist. Hence, there needs to be a certain amount of cost involved to dispose these aggregates.
Advantages of bio-mining
So with waste disposal becoming a critical area for communities around the world, and with the Indian government now mandating bio-mining and bioremediation, let’s look at the advantages:
✦ The process generates near zero emissions and leaves near zero residues.
✦ Some of the aggregates generated from the process like refuse-derived fuel can be reused, recycled or repurposed, thus exerting less pressure on the economy to use virgin natural resources like fossil fuels.
✦ The process puts a permanent end to the source of pollution and hence the degradation of underground water resource, surface water and soil contamination stops.
✦ With the dumpsite vanishing, the residents staying close by can breathe a sigh of relief; no more bad odour.
✦ The complete land which is reclaimed can be put to alternate use especially to develop facilities in better management of solid waste, ensuring another landfill does not come up at the mining site.
✦ Greenhouse gas emissions reduce significantly.
✦ Landfills are a breeding ground for flies and mosquitos. Clearing the landfills can help immensely decrease the prevalence of vector-borne diseases.
Zigma benchmarks bio-mining processes
In March 2015, the five co-founders of Zigma came together with one objective to do something of social value in the field of waste management. This was around the same time that Prime Minister Narendra Modi had announced the Swachh Bharat mission with an aim to make India clean for future generation.
“In keeping with the spirit of Make in India, which the government was aggressively promoting at the time, Zigma designed, fabricated and financed its own mobile MSW plant with the help of its inhouse engineering team who were hired from different industries,” says Dharmaraj
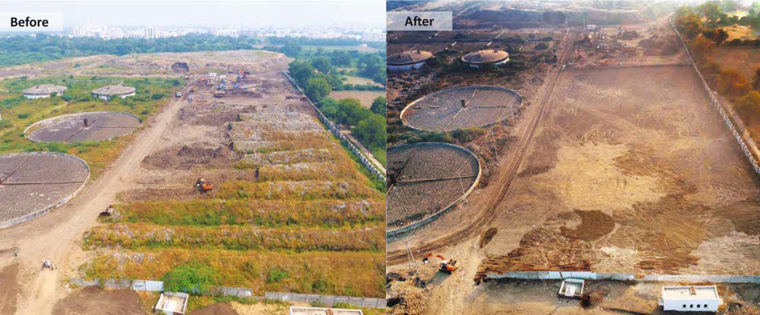
A decade ago, India’s fresh waste processing plant dumped waste termed as “rejects” in the landfill sites which turned out to be also responsible for these giant landfills standing tall today. Zigma wanted to customise the machine to ensure that there was fine segregation that can support near zero concept. It therefore did not opt for machines that did not offer this. Armed with its very own mobile MSW plant, Zigma embarked on its first project in Kumbakonam, Tamil Nadu, which required reclamation of a 12-acre dumpsite, filled with 1,10,000 cubic meters of solid waste. In just six months, the enterprise cleared over 60,000 metric tonne of waste, reclaiming
a remarkable 3.5 acre of land. The project was a first-ofits kind integrated model with zero residues tried anywhere in India. A team from Supreme Court Panel Members and Ministry of Housing and Urban Affairs visited the plant, which was featured in the SBM Best Practices. Following this, the project was showcased in the first Swachh Sarvekshan in 2016, recommending all the Urban and Local Bodies to implement a similar model in their respective dumpsites.
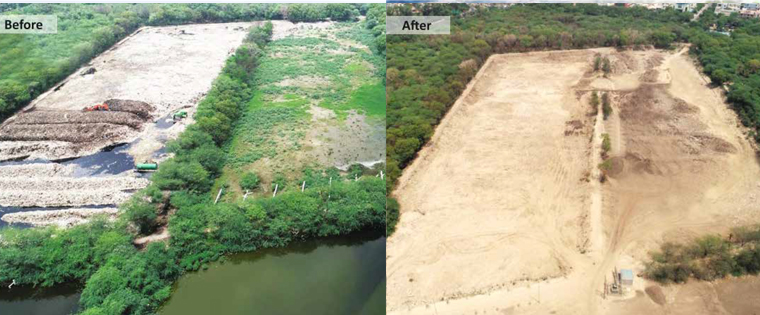
Since then, the company has gone from strength to strength and has been introducing the integrated landfill mining model to the ULBs. “It is like the dumpsite is outsourced to us wherein we take care of everything―setting up the plant, stabilisation of waste, segregating the waste with state-of-the-art plants and then recycling, reusing or, in some cases, finding other uses for the aggregates generated,” says Dharmaraj.
The three Ts
Zigma has been introducing a holistic approach in the Indian Solid Waste Management sector which was looking for an efficient solution with transparency. Zigma follows the three Ts―technology, transparency and testing―n their operations. “The scalability, efficiency and technology of our machines are responsible for the disposal of aggregates,” says Dharmaraj. “We hence lay high emphasis on continuous research, development and innovation.”
Dharmaraj believes that transparency in operations iswhat makes them stand apart from competition. The company has designed its own ERP system which provides live data feed to the ULBs. This includes 24x7 video monitoring of operations, automated weighbridge data live update with unique data-like truck picture capturing. Further, a system is incorporated which provides aerial view of reclamation of the land at every stage, captured using drones.
“All the officials concerned can access the information anytime and monitor the dumpsite 24x7. Transparency and traceability of the materials were almost non-existent earlier and Zigma has benchmarked this in the landfill mining projects in India, being the first organisation to implement this procedure. Traceability― where you send these aggregates very important and needs to be monitored,” says Rajasekaran. “Otherwise, the risk of contamination that is now limited to the dumpsite can go offsite.”
In 2016, Zigma tied up with a South Korean landfill mining major company Forcebel, which has cleared more than a hundred landfill sites across the world. The collaboration with Forcebel was envisioned for developing the most advanced landfill mining system in the world, with a very high focus on quality, scalability and technology in India. Zigma uses its expertise in implementing some of the best practices in landfill mining and implementing projects in extreme situations like in lakes and river sides or in low temperatures. Forcebel’s reclaimed sites across the world have been converted into residential complexes, subway stations and football stadiums among others. The collaboration was a feather in the cap and helped Zigma upgrade its already customised efficient segregation systems to ensure flawless quality of aggregates and achieve its vision of achieving near zero residues.
Zigma lays high emphasis on its testing of aggregates before disposal and has worked its way in achieving the same. The company collaborated with reputed institutes like IIT, Mumbai to undertake research and provide advisory in methodologies for aggregate disposal. Zigma now has tie-ups with various laboratories across the country with NABL and MoEF accreditation to undertake various tests. These tests are carried out in strict adherence to SWM Rules and CPCB Guidelines. In addition, the company ensures an in-house laboratory being set up at every project site for certain tests that need to be carried out before everyday disposal of aggregates.
“There were no standards for landfill mining and bioremediation earlier,” says Rajasekaran. “But the government―both at the Centre and the states―is very proactive, and a lot of initiatives are being taken up, including the CPCB guidelines to ensure that responsible disposal has been undertaken.” Why is testing so important? Dharmaraj elucidates, “Testing not only confirms whether the aggregates are safe to dispose but also allows to decide if any further treatment is required before the same can be disposed offsite.”
One of the aggregates which is a segregated combustible fraction, consisting of low value/non-recyclable plastics, cloth, bags, polyester material, tyres, rubber and wood, is converted into refuse-derived fuel and sent to cement plants to be used as alternate fuel (as a replacement to coal) or to Waste to Energy plants to incinerate and produce energy.
The company has grown in a sustainable manner from 2016. It began with 30 people and today employs more than a thousand people. As of now, Zigma has cleared over 25 lakh tonne of legacy waste and cleared over 125 acres of land which is equivalent to about 1.69 million tonne of carbon dioxide emissions saved.
Also, the refuse-derived fuel generated from the dumpsites has successfully replaced fossil fuel and helped save 45,000 tonne of carbon dioxide emission, which is equivalent to carbon sequestered by 59,254 acres of US forests in one year. For Zigma, it is not only important to remove and process the waste but also using the waste to replace depleting commodities like fossil fuels and reduce greenhouse gas emissions.
The recovery cost of a 45-acre land in Vijayawada is pegged to be around Rs600 crore, which is considered to be at least 20 times higher than the bio-mining project cost.
The Ataldara dumpsite recovery in Vadodara was appreciated as one of the best projects, considering its cost saving of 250 crore to the Government, and eradicating the pollution problem of the Vishwamitra river due to the existence of this 20-year-old dumpsite.
Zigma has also reclaimed dumpsites near water bodies like lakes and rivers and sites in the centre of cities. At present, it is operating with 18 projects in 5 different states. It was awarded the Best Innovation in Sustainable Solid Waste Management Award by Frost and Sullivan in 2016. In 2020, it was awarded by CII 3R Award for Excellence in Municipal Solid Waste Management Award.
The way forward
With legacy waste and dumpsites becoming a huge, stinking problem for India, what is essential is making landfill mining of legacy waste more professional to envisage a landfill-free nation. Rapid urbanisation and population explosion have put extreme pressure on the Indian economy. Technologies like landfill mining could be used to implement setting up of large infrastructure projects like stadiums, bus terminals and residential complexes over the landlocked landfill sites, which are currently an eyesore and continuous source of pollution. Such ideas can also attract private players for amortisation of these lands to fund the landfill mining activities, thus saving the government treasury funds.
Being a signatory to the Paris Climate Summit, India is showing a lot of willingness in implementing dumpsite reclamation project.
📣 The Week is now on Telegram. Click here to join our channel (@TheWeekmagazine) and stay updated with the latest headlines
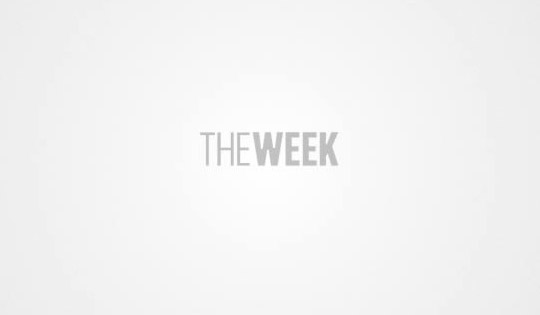
At collapsed Baltimore bridge, focus shifts to the weighty job of removing the massive structure
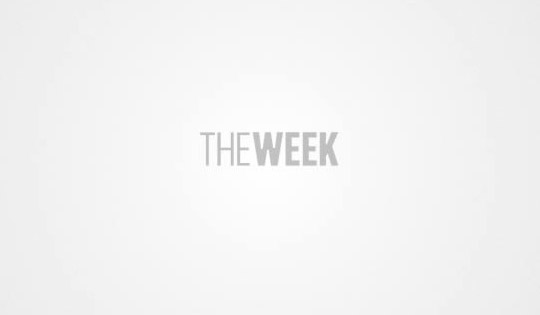
ED files first chargesheet in Delhi Jal Board money-laundering case
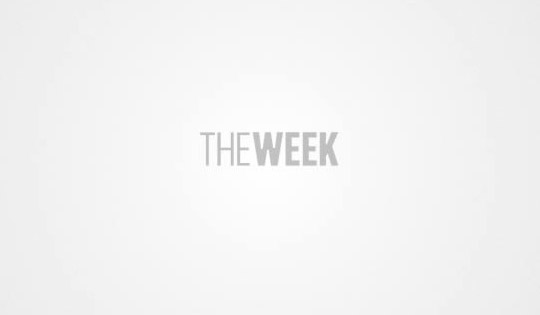
Actor Daniel Balaji passes away due to heart attack
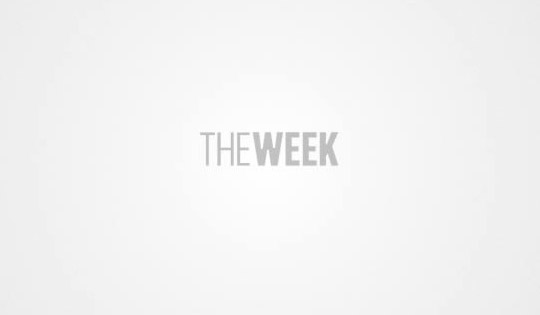
LSG's captaincy change explained: Why Nicholas Pooran replaced KL Rahul ahead of Punjab Kings game?
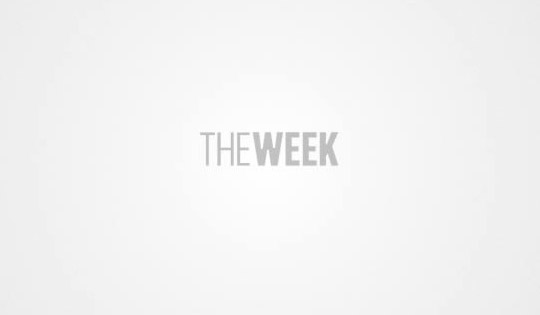
Neuroscientists uncover mechanism for memory consolidation
Editor's pick.
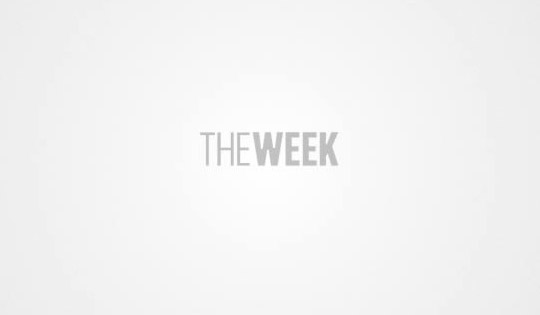
Exclusive: 'People are casting their votes only in Modi's name', says Rajnath Singh
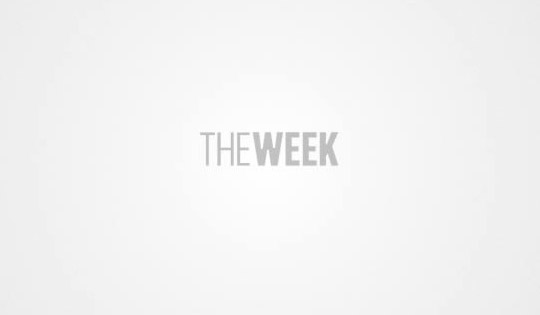
'Malayalam cinema is yet to discover lot of untapped markets': Prithviraj
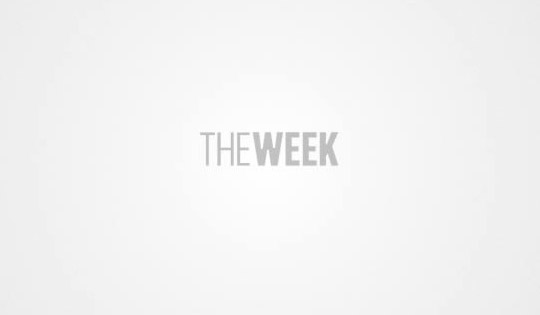
Meet the doctors who have turned their passions into alternative professions
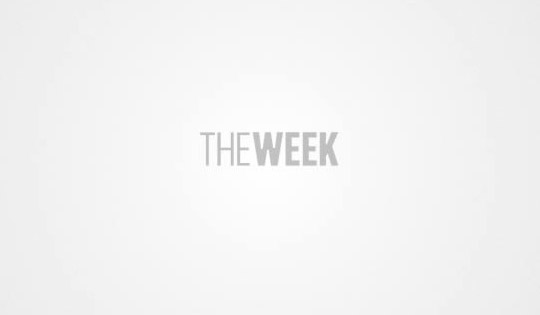
Equity surge

An official website of the United States government
The .gov means it’s official. Federal government websites often end in .gov or .mil. Before sharing sensitive information, make sure you’re on a federal government site.
The site is secure. The https:// ensures that you are connecting to the official website and that any information you provide is encrypted and transmitted securely.
- Publications
- Account settings
Preview improvements coming to the PMC website in October 2024. Learn More or Try it out now .
- Advanced Search
- Journal List
- J Environ Health Sci Eng
- v.19(1); 2021 Jun
Estimating greenhouse emissions from sanitary landfills using Land-GEM and IPCC model based on realistic scenarios of different urban areas: a case study of Iran
Mehdi ahmadi moghadam.
1 Environmental Technologies Research Center, School of Health, Ahvaz Jundishapur University of Medical Sciences, Ahvaz, Iran
Rozhan Feizi
2 Department of Environmental Health, Behbahan Faculty of Medical Sciences, Behbahan, Iran
Masoud Panahi Fard
3 Student Research Committee, School of Health Ahvaz, Jundishapur University of Medical Sciences, Ahvaz, Iran
Neematollah Jaafarzadeh Haghighi Fard
4 Environmental Research Institute, Tehran University of Medical Sciences, Tehran, Iran
5 Toxicology Research Center, Ahvaz Jundishapur University of Medical Sciences, Ahvaz, Iran
Maryam Omidinasab
6 Department of Environmental Health Engineering, Abadan Faculty of Medical Sciences, Abadan, Iran
Maryam Faraji
7 Environmental Health Engineering Research Center, Kerman University of Medical Sciences, Kerman, Iran
Bamshad Shenavar
8 Khouzestan Department of Environment, Ahvaz, Iran
This study examined the emission of greenhouse gases from municipal solid waste disposal centers in different regions of Iran based on different scenarios. Assuming landfill site opening in 2012 and considering the 20-year plan period for its usage, the amount of wastes entering the landfill site was calculated for 2012–2032. For calculating the production of methane (CH 4 ) and other gases during different years of the project, Land-GEM and IPCC model were used. We defined 9 scenarios for these two models based on the growth rate of population and waste generation. The results revealed that the lowest amount of gas emission in nine scenarios by Land- GEM model was related to non-methane organic compounds (NMOCs). According to the results obtained, the total emissions of greenhouse gases from sanitary landfills for Iran in 2032 were 3,844,000 Mg/year, the largest and lowest amounts of gas emission were related to Tehran region, 860,400 Mg/year, and the South Coast area of the country,138,200 Mg/year, respectively. The major section of the gas production in both landfills was related to greenhouse gas and carbon dioxide emissions. The difference in gas production in the studied regions was due to differences in the percentage of moisture and organic compounds.
Introduction
In recent decades, global warming has become a serious environmental problem that threatens the world. This has occurred due to greenhouse gaseous emissions such as CO 2 , CH 4 , and N 2 O from anthropogenic activities. The developing countries in 2000 were responsible for the emission of 29 % of greenhouse gases, with this amount estimated to reach 64 % and 76 % in 2030 and 2050, respectively [ 1 , 2 ]. Methane gas (CH 4 ) is one of the most important greenhouse gases (GHG) with a high heating value, which has the high potential for global warming (25 to 30 times more than CO 2 ). In addition, the rate of methane emission was 18 % of total greenhouse gases and the second rank compared with the other gases[ 3 , 4 ]. Also, if its amount reaches 5 to 15 % of the air volume, it will explode and cause damage. As landfill is the most common method for waste disposal, so these centers are known as the main sources of CH 4 emissions. The anaerobic decomposition of waste in the landfill via microorganisms leads to the release of CH 4 gas and other gases [ 5 ]. On the other hand, CH 4 has a very high thermal value, and thus is economically important. Hence, according to the mentioned reasons, control and collection of CH 4, emissions is essential. Different methods such as the evaluation site, triangular model, the stoichiometric, the Intergovernmental Panel on Climate Change (IPCC), and mathematical modeling exist for estimating the amount of gas emission [ 6 , 7 ]. Various mathematical models have been developed worldwide, where landfill gas emissions (Land-GEM) model is the most important and most flexible [ 8 ]. In this study, IPCC and Land-GEM models were used. These models were proposed by the US Environmental Protection Agency and provide a very accurate estimate of the amount of CH 4 produced in different years [ 9 , 10 ]. Worldwide, numerous studies have been carried out to determine the amount of CH 4 gas production and other gases in sanitary waste disposal centers, while studies conducted using the Land-GEM software and IPCC are sparse. Talaiekhozan et al. (2016) evaluated and analyzed gaseous emission such as carbon dioxide and methane in a landfill area using Land-GEM software in Rodan. Their study results showed that only 24.18 % of the waste produced in Rodan is perishable and the production rates of methane and carbon dioxide would be equal to 112 and 308 tons per year respectively in 2019 [ 11 ]. Amirmahani et al. (2020) estimated methane gas generation rate from Kerman City landfill using Land-GEM software and reported that methane gas produced in this landfill in 2015, 2021, 2027, and 2033 would be roughly 72, 2,540, 3,914, and 5,015 m 3 h − 1 [ 12 ]. Fallahizadeh et al. (2019) reported that in Yasuj, the municipal solid waste landfill methane gas produced during the years 2009, 2010, 2011, and 2012 was obtained to be 250, 275, 303, and 330 m 3 /h, respectively[ 13 ]. Jigar et al. (2014) using IPCC model for estimating methane gas emission from municipal solid waste landfill showed that the net Annual emission value from solid waste landfills was 7.11 (Gg yr-1) in 2003, while the maximum methane production rate by the IPCC model was calculated to be 9.98 (Gg yr-1 ) observed during 2012 [ 14 ]. In Iran, no comprehensive study has been carried out with this scope to calculate the amount of GHG emission in waste disposal centers. Carbon dioxide and methane are the two main gases formed in landfills, which are also considered as greenhouse gases. Due to the different characteristics of the wastes in different regions and the compatibility of Land-GEM and IPCC models with these characteristics, accurate estimates of the production of these gases can be presented. Thus, this study aimed to determine the amount of GHG emissions from municipal solid waste disposal centers in different regions of Iran considering the 20-year plan period based on nine scenarios using a Land-GEM simulation model and IPCC. This study was the first study to estimate the amount of GHG production in landfill sites across different regions of Iran, whose results can be used to plan for energy generation and other applications of gases.
The study area
Iran with 1,648,195 square kilometers area is located in the northern hemisphere, west of Asia, and in the western part of Iran’s plateau. It has 31 provinces, divided into 11 geographic regions in the current study, with a population of more than 80 million in 2018. The needed data included of population, waste generation per capita, and solid waste components, moisture content, etc. Were gathered some from 31 provinces and at the end, the country classified to eleven target regions. The mentioned eleven regions are shown on the map of Iran in Fig. 1 .

The map of eleven regions of Iran
Description of the scenario
This study has been based on nine scenarios including per capita of ordinary waste generation (kg/day/person), enhancement policies (assuming 1.5 % increase in per capita of waste generation), and reduction policies (assuming 3 % decline in per capita of waste generation). By considering the above scenarios, the GHG emissions were investigated. The checklist of scenarios is described in Table Table1. 1 . The differences in population growth rate is related to use of three different scenarios. The first scenario is related to the increase in population growth rate, which has been selected according to the government’s policies in Iran and the emphasis on population growth. The second scenario is related to the real population growth rate in the last 10 years, and the third scenario is based on predictions that some studies have estimated the growth rate will decrease to 0.87 cause of the economics conditions and social awarrances. Also, the results of reviewing data from different provinces of Iran showed that the amount of Waste generation can fluctuate from a 3 % decline to a 1.5 % increase. The per capita of waste generation (kg/day. Person) for each region during 2012 is reported in Table Table2 2 .
Various scenarios in this study
Per capita of waste generation in 11 regions in 2012 [ 15 ]
For this research and calculation of GHG production during different years of the project, Land-GEM software and IPCC model were used. According to the mathematical equation used in these models, some information is necessary such as the amount of waste in the landfill site, number of years of exploitation, CH 4 production potential, and production constant of methane. Assuming opening landfill site in 2012 and by considering the 20-year plan for its usage, the amount of waste entering the landfill was calculated in 2012–2032. In Land-GEM software, the productions of four major pollutants including CH 4 , carbon dioxide (CO 2 ), total gas, and non-methane organic compounds were calculated and the results are presented in the form of a figure and table.
Land –GEM model
Using Land-GEM software, the amount of CH 4 gas produced (Mg/year) was estimated. The general form of the formula is as follows: Eq. ( 1 ) [ 10 , 16 ]
Q CH4 : Methane production in a year (m 3 /year).
I: The firstly year of entering waste in the landfill.
N: The lastly year of entering waste in the landfill - The firstly year of entering waste in the landfill.
J: Intervals of 1 year.
K: Methane production rate (year).
L 0 : Methane production potential (Mg/m 3 ).
M i : The amount of the waste accepted by the landfill site in the year I (Mg).
T ij : The lifetime of the j waste section has been accepted, in the year i.
In this software, the default values for the production constant (k) were 0.05 and the inertial power of gas production was 100 (L 0 ) [ 17 ].
Model inputs are:
- Landfill site history.
- Waste acceptance rate.
- The concentration of non-methane volatile organic matter.
- CH 4 production constant (Based on the first-order relationship for organic matter decomposition).
- CH 4 gas production potential.
- The concentration of toxic pollutants.
IPCC model is a simple method for estimating CH 4 emissions from solid waste disposal sites during the period used in landfills.
- A) The default IPCC methodology is based on the theoretical gas yield (a mass balance equation).
- B) Theoretical first-order kinetic methodologies, through which the IPCC guidelines introduce the “First order decay model” (FOD) [ 14 ].
The methodology for estimating CH 4 emissions from SWDS in the revised IPCC (2006) guidelines are based on the FOD method [ 18 ].
The formula for calculating methane emission from sanitary landfill is as follows [ 19 , 20 ]:
A = CH 4 production (Mg/ year).
X = Year in which waste was disposed.
S = Start year of inventory calculation.
T = Inventory year for which emissions are calculated.
W x = the quantity of waste disposed at the solid waste disposal site (Mg).
L = CH 4 production potential (Mg CH 4 /Mg waste).
=MCF×DOC×DOC F ×F×16/12[IPCC nomenclature].
=L 0 × 16/0.02367 × 10 − 6 .
L 0 = CH 4 production potential (m 3 CH 4 /Mg waste) [AP-42 nomenclature].
MCF = CH 4 correction factor (fraction), typically 1 for managed landfills.
DOC = Degradable organic carbon [fraction (Mg C in waste /Mg waste)]
DOC F = fraction of DOC decomposed (fraction), generally assumed to be 0.5.
F = fraction by volume of CH 4 in landfill gas generally assumed to be 0.5.
K = decay rate constant (year − 1 ).
Population calculation during the project period
The population data used in this research were obtained from the statistics center of Iran. According to this information, the growth rate of the population is about 1.29 % per year. Also, assuming a decline of 0.87 % and an increase of 1.9 % in the population growth rate, the population of different years of the plan period was estimated.
To calculate the population in different years during the plan period, the following formula was used [ 21 ].
P n : The population at time t.
P 0 : The population at time 0.
γ: The population growth rate.
n: A period (year).
Results and discussion
According to the amount of methane gas calculated using Land–GEM model, it is possible to design and implement CH 4 collection systems for every landfill site. The advantages of this system include use of CH 4 gas, reducing gas accumulation in landfills, preventing explosion at landfills, and preventing leakage of gas to the aquifer. By calculating the population of different regions and per capita of waste generation in the different years of the plan period, the amount of generation waste was obtained. The amount of solid waste entering the landfill site in different areas as (Ton/year) is shown in Table Table3. 3 . According to the results obtained, it is clear that in 2012 to 2032, the maximum amount of waste entering to the landfill site is related to Tehran with an initial population of 16,119,801, while the lowest amount of entrance waste is related to the Persian Gulf coast with an initial population of 2,611,132. The reasons for the difference in the amount of waste generation in the different areas of the country are different populations, economic conditions, commercial activity, and a different consumption pattern causing varying rates of waste generation [ 22 , 23 ]. In this study, using Land- GEM software, the amount of CH 4 and other gases in the landfill site were determined by the graph. Due to the extensive difference between the two regions, Tehran and the Persian Gulf coast, regarding the highest and lowest quantity of population and the quantity of waste generation, the results obtained from the model for the two regions are shown in Fig. 2 (a-c). This figure shows the rate of gas production in the landfill site for over 20 years. As expected, the amount of CH 4 , CO 2 , and total gas production increased in the course of utilizing the landfill site, and in the final years of the project it reached the maximum amount. The highest rate of gas production was related to 2022–2032, but after the end of the plan period, the curve slope decreased. During this time, gas production continued after the landfill site closure for many years and had a major contribution to greenhouse gas emissions. According to scenario 9, in Tehran region, the amount of emissions of total greenhouse gases and CH 4 in 2032 was obtained 860,400 and 229,800 Mg/year, respectively, and in the Persian Gulf coast the quantity emissions reached 165,500 and 44,200 Mg/year, respectively. Also, the lowest amount of gas production was related to scenario 1, where for Tehran area, total emissions of GHG and CH 4 gas were obtained 709,600 and 189,500, and in the Persian Gulf coast area reached 138,200 and 36,930 Mg/year, respectively. It can be concluded that a major part of the production of gas in both landfills was related to GHG and carbon dioxide emissions. The difference in the amount of gas produced in different regions was due to the variations in the percentage of moisture and the organic compounds [ 24 ]. Given the fact that about 70 % of materials entering landfill sites are biodegradable [ 25 ], hence, in the first years, the decomposition rate is high. The high slope of the graphs indicates the correctness of this fact. In the following years, gradually with the arrival of new wastes to the landfill and considering the down rate in the production of gas in the previous waste, the rate of degradation of waste and as a result, the slope of the chart decreased [ 26 ]. After 2032, as indicated in the graphs, because no waste i.a. entered into the landfill site, the speed of gas production reached its lowest. During this time, the production of all three types of gas continues for many years. In the scenario of reducing the waste generation, it was assumed that between 40 % and 50 % of materials, such as plastics, paper, and plastics from the landfill site are removed and recycled, and 40 % of organic materials are composted, hence the amount of pollution and GHG emission was reduced [ 27 – 29 ]. In the scenario of increasing waste generation, it was assumed that the recycling rate is less than 30 %. On the other hand, some factors such as the economic development, changing consumption patterns, improving living standards, and increasing urban populations lead to increased waste generation, hence the amount input garbage to landfill site and gases rate production increased [ 30 – 32 ]. In addition, various factors have positive and negative effects on gas emissions. The rate and volume of produced gases depended to the composition of waste, moisture, geographical conditions, the amount of waste leachate, the presence of oxygen, and population growth; thus, comparing the results of this study with other similar studies is not reasonable [ 33 , 34 ]. As in Table Table4 4 identified, the highest quantity amount of gas release in 2032 in all scenarios was related to total greenhouse gases (GHG) and the lowest amount was related to NMOCs gas respectively. Specifically, the release rate of NMOCs in this study is about 1 % of total gas emissions. In other words, NMOC concentration depends on the type of waste in the landfill and the reaction of different compounds during the anaerobic degradation of the waste [ 21 ]. Using IPCC model, the value annual CH 4 emission in regions of Tehran and Persian Gulf coast was compared. The results obtained in Fig. 3 , showed that emission of CH 4 for Tehran region in scenario 1 rose from 5214.3 tons in 2013 to 6147.1 tons in 2032, and in scenario 9 from 5649.8 tons to 8078.8 ton. Also, in the Persian Gulf coast region, the amount of emission methane in scenario 1 was elevated from 1152 tons in 2013 to 1358.2 tons in 2032, and in scenario 9 from 1244.5 tons to 1779.5 tons. The results of a study conducted by Kalantarifard et al. to determine CH 4 generated from the landfill site revealed that the value of methane emission in 2012 reached 443.60 Mg/year while in 2032 it decreased to 1.74 Mg/year. In addition, the maximum amount of CH 4 emissions was about 4170 Mg/year during 2012–2015 [ 35 ]. GHG emission identified in Tunisian landfill site, one year after the landfill closure in 2011, maximum gas production was reached (2.61 × 10 7 m 3 /year). In another study performed on the emission of GHG from a landfill site by Sing and et al. (2016) in Delhi India, the results showed that urban waste disposal is the most important source of GHG emission. Also, the results obtained showed that CH 4 emissions from Okhla Ghazipur and Bhalswa Landfills for the year 2011–2012 were 77.42 Gg/ year, 3845.20 Gg/ year, and 91.23 Gg/ year by Default Method; and 52.60 Gg/ year, 2611.99 Gg/ year and 61.97 Gg/ year by First -Order Decay method [ 36 ]. The results obtained from a study done by Chalvatzaki and Lazaridis (2010) on GHG emissions from landfill site showed the biogas production rate by the Land-GEM model during 2008 for A phase was 1.64 × 10 3 Mg/ year, and for B phase during 2014 the biogas production rate was 2.70 × 10 3 Mg/year [ 6 ]. Weitz and et al. (2012) By using of IPCC model, estimated that methane emissions from solid waste landfill in Panama for the years 1990–2020 was from 24.6 Gg in 1990 to 41.6 Gg in 2020 [ 37 ]. The results obtained from a study done by Rezaee and et al. (2013) by using Land-GEM software, demonstrate methane, carbon dioxide, and non-methane organic compounds emissions from Sanandaj were 6184, 16,970, and 266 tons/year, respectively [ 34 ].
The solid waste entering the landfill site in different scenarios (Ton/year)
The gases generated in the landfill site in 2032(Mg/year)

The amount of exhaust gases from the landfill site for two regions of Tehran and the Persian Gulf coast ( a ) per capita decline ( b ) per capita ordinary ( c ) per capita increase

Comparison of methane emission in Tehran and Persian Gulf coast landfill site in 2013 and 2032
Conclusions
This study provided information on estimating greenhouse emissions from sanitary landfills using Land-GEM and IPCC model based on realistic scenarios of different urban areas in Iran. The results obtained from these models showed that a significant amount of gas was produced in the first years of waste entrance to the landfill, with main landfill gases being CO 2 and CH 4 , both of which are identified as GHG. Because greenhouse gases from human activities such as deforestation, waste disposal, agriculture, industry, and especially fossil fuels are the main reasons for climate change, it is important to identify landfill gases and estimate emissions rate. As a result, the use of Land-GEM and IPCC models can be useful for estimating the maximum amount of production gases. Also, by applying of methane production calculation we be able to predict and designed gas collection systems for each landfill site. At the end, The results of this study help domestic policymakers to make appropriate decisions for municipal waste management to control and minimize the parameters affecting the production of greenhouse gases in sanitary landfills. Given that the consequences of increasing global greenhouse gases emissions are not limited to geographical and political boundaries, the findings of this study can provide background information to increase the attention and challenge in other countries to improve the municipal waste management system.
Acknowledgements
This work was supported by the Institute for Environmental Research, Tehran University of Medical Science (Grant No. 23313-46-02-92).
Declarations
The authors declare that they have no conflict of interest regarding the publication of the current article.
Publisher’s note
Springer Nature remains neutral with regard to jurisdictional claims in published maps and institutional affiliations.
- Ground Reports
- 50-Word Edit
- National Interest
- Campus Voice
- Security Code
- Off The Cuff
- Democracy Wall
- Around Town
- PastForward
- In Pictures
- Last Laughs
- ThePrint Essential

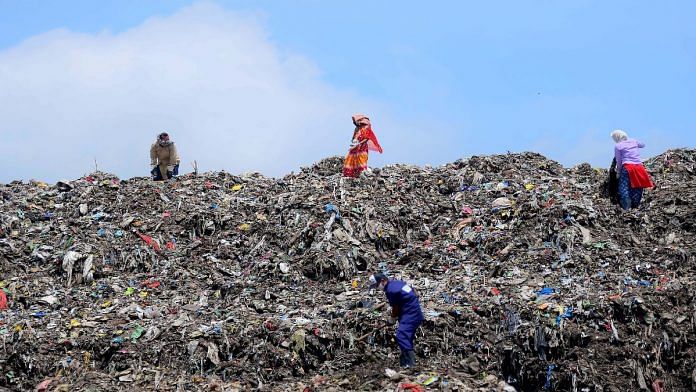
New Delhi: Three Indian cities, each located in a different state, have set a major benchmark for the rest of the country when it comes to ideal waste management practices, according to a new NITI Aayog report.
At a time when garbage disposal sites have become a massive problem for most urban centres, Chhattisgarh’s Ambikapur, Maharashtra’s Chandrapur and Kerala’s Taliparamba have adopted a “zero-landfill model” of development, which seeks to phase out dependency on new landfills. This achievement has landed the three cities in a list of 28 with the best waste management practices, mentioned in the report released by NITI Aayog, in collaboration with the Centre for Science and Environment, Monday.
The report was launched in the presence of NITI Aayog vice chairperson Dr Rajiv Kumar, CEO Amitabh Kant, special secretary Dr K Rajeswara Rao, and CSE director general Sunita Narain.
“With rapid population growth in urban areas, capacities of local authorities often fall short of achieving the set goals of urban service delivery. Therefore, sector stakeholders must be equipped with adequate knowledge resources to plan efficient waste management systems,” said Kant at the launch.
According to the report, a zero-landfill model “is based on resource recovery and principles of circular economy” that are socially, environmentally, and economically sound. It identifies a “zero-landfill city” as one that ensures maximum quantities of waste are subjected to scientific treatment and recycling, thus reducing the amount of residual solid waste and minimising the need to construct new landfills.
“It is a holistic and multi-stakeholder approach that ensures that waste is segregated at the source itself, recyclables are extracted and channelized to the recycling industries for various gainful applications, and biodegradable waste is treated in a decentralised manner,” the report says.
On Ambikapur, the report says that with the “intervention of the local administration and women self-help groups” and “inspired by the concept of the Garbage Clinic Model”, the city is now able to achieve 100 per cent segregation, collection and processing of waste.
Also Read: Only 9 per cent of plastic waste is recycled. Seeing its potential as a product can change that
Source segregation: Cities that stood out
Titled ‘Waste-wise Cities: Best practices in municipal solid waste management’ , the NITI Aayog report shortlists cities based on 10 parameters: source segregation, biodegradable waste management, material processing, plastic waste management, construction and demolition (C & D) waste management, sanitary waste management, landfill management, technological innovation and e-waste management.
These aspects in particular were chosen because they are part of a “a sustainable value chain” and include practices that other cities can benefit from too.
On source segregation, “a fundamental and non-negotiable condition for a sustainable waste management,” Allappuzha (Kerala), Indore (Madhya Pradesh) and Panaji (Goa) scored highest out of the 28 cities.
The report calls Indore the “number one city in the waste management sector in India”, stating that it has “a robust communications strategy to bring about behavioural change at the mass level”. Its aim to motivate citizens to embrace segregation is backed up by “a robust monitoring system and enforcement through a series of by-laws”. Its success lies in a combination of “source segregation, participation of all stakeholders and good governance”, the report adds.
When it comes to processing materials found in the waste, after it has been collected from the source, Bhopal (Madhya Pradesh), Surat (Gujarat), Jamshedpur (Jharkhand) and Dhenkanal (Odisha) imbibed the best practices, according to the report.
The cities to perform best in plastic waste management were Bicholim (Goa), Gangtok (Sikkim) and Kumbakonam (Tamil Nadu), while North Delhi (Delhi) and Gurugram (Haryana) performed best when dealing with C&D waste.
Sanitary waste: ‘Breaking the taboo’
“Breaking the taboo” around sanitary waste, the city of Karad in Maharashtra has managed to achieve a “100 per cent collection rate” in this category.
Sanitary waste management has been “the least explored and debated of all the streams of solid waste that is generated at the household level”, the report says.
Explaining the modus operandi behind Karad’s success, the NITI Aayog report says that the city administration ensures that sanitary waste “is transported and processed separately in the local Common Biomedical Waste Treatment Facility (CBWTF)”.
“All this was achieved through “minimal investment on infrastructure and higher accountability amongst citizens and city government through a combination of communication and enforcement strategy,” it adds.
Pune too did well in this regard, with the administration currently “in the process of exploring a state-of-the-art technology to make value added products from their sanitary waste”.
The cities of Bobbili (Andhra Pradesh), Mysuru (Karnataka) and Vengurla (Maharashtra) found mention for handling biodegradable waste. Zero waste plants in Mysuru, in particular, scripted its success.
Using tech to solve the problem
Leh (Ladakh) installed a 30 tonne solar waste segregation plant, earning its spot among the cities that use technology to solve waste management. Kakinada (Andhra Pradesh) and Bengaluru (Karnataka) also find place in the list for using ‘Radio Frequency Identification’ for garbage collection.
Keonjhar (Odisha) and Vijaywada (Andhra Pradesh) are also among the cities to use tech in waste management, incorporating real time tracking systems, according to the report.
On innovation and e-waste, the cities to perform best were Paradeep (Odisha), Panchgani (Maharashtra), Thiruvananthapuram (Kerala) and Jamshedpur (Jharkhand).
(Edited by Gitanjali Das)
Also Read: What India can learn from this scenic Kerala town about waste management
Subscribe to our channels on YouTube , Telegram & WhatsApp
Support Our Journalism
India needs fair, non-hyphenated and questioning journalism, packed with on-ground reporting. ThePrint – with exceptional reporters, columnists and editors – is doing just that.
Sustaining this needs support from wonderful readers like you.
Whether you live in India or overseas, you can take a paid subscription by clicking here .
- environment
- Solid waste management
LEAVE A REPLY Cancel
Save my name, email, and website in this browser for the next time I comment.
Most Popular
Ed reply to kejriwal plea against arrest: ‘didn’t oppose remand, can’t disregard approver at probe stage’, ‘upright’, 26/11 hero & expert from terror to financial fraud: who is sadanand date, new chief of nia, 64 indians fly to israel ‘under g2g agreement’ to fill construction job vacancies left by palestinians.

Required fields are marked *
Copyright © 2024 Printline Media Pvt. Ltd. All rights reserved.
- Terms of Use
- Privacy Policy
A study on contamination of ground and surface water bodies by leachate leakage from a landfill in Bangalore, India
- Original Research
- Open access
- Published: 12 December 2018
- Volume 9 , article number 27 , ( 2018 )
Cite this article
You have full access to this open access article
- B. P. Naveen ORCID: orcid.org/0000-0002-7085-4912 1 ,
- J. Sumalatha 2 &
- R. K. Malik 1
20k Accesses
63 Citations
Explore all metrics
This paper discusses the effects of a potential leachate leakage from a municipal solid waste landfill, situated at Mavallipura, Bangalore, India, on the surrounding water bodies. The landfill area is spread over an area of about 100 acres that began accepting waste from 2005. MSW was deposited in non-engineered manner that has resulting in steep and unstable slopes, leachate accumulation within the MSW mass, and leachate runoff into nearby water bodies such as ponds and open wells. The current study investigates the physicochemical characterization of landfill leachate and nearby water bodies. The batch leach tests were conducted to know the heavy metal concentrations in the contaminated soil. A series of column tests were also conducted to estimate the migration rates of different contaminants through the soil. Furthermore, these transport parameters were considered as input for fluidyn-POLLUSOL model to estimate the migration of leachate from the landfill site to the surrounding water bodies.
Similar content being viewed by others
Investigation of Sub-surface Contamination Around the Landfill Site: A Case Study
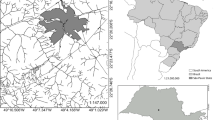
Temporal behavior analysis of leachate contamination in a tropical landfill
Gabriel Messias Moura de Faria & Giulliana Mondelli
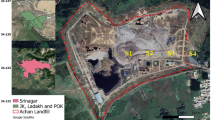
Landfill leachate a new threat to water quality: a case study from the Temperate Himalayas
Shayesta Islam, Haleema Bano, … Owais Ali Wani
Avoid common mistakes on your manuscript.
Introduction
Generally, the municipal solid waste landfills create lots of environmental pollution due to landfill gas combustion, leakage of leachate and foul smells. Among all these, leakage of leachate affects the surrounding environment the most, especially the surface and ground water bodies because the leachate consists of high concentrations of heavy metals, organic compounds and toxic contents. Recently, several cases have been reported around the world related to pollution of water bodies which were caused by municipal solid waste landfills [ 1 ].
The production and usage of heavy metals such as copper, cadmium and zinc have increased substantially over the years [ 2 ]. The excess quantity of heavy metals disposed off the land can cause significant damage to the environment and human health as a result of their mobility, solubility and their ability to transfer in water or plants [ 2 , 3 ].
The leachate from MSW landfills may leak into groundwater aquifers due to rainfalls, spread into the adjacent river system by groundwater flow and pollute the surrounding environment. However, this process does not stop even after the landfill activities have stopped receiving solid waste. Hence, it is very essential to keep assessing and monitoring the surroundings of decommissioned landfill sites. The contaminant transport must be modelled in soil and water media to estimate the level of contamination that has happened/going to be happening. Furthermore, these models are helpful in selecting a proper remediation method for the affected site.
The contaminant transport parameters can be determined through laboratory column experiments to get the accurate values or they can be estimated from the related data documented in the literature by using some correlations. The contaminant transport parameter values for different metal ions are available in the previous studies [ 4 , 5 , 6 , 7 , 8 , 9 , 10 , 11 , 12 , 13 , 14 , 15 , 16 , 17 ].
Currently, various specialized computer software packages have been evolved and used to address the contaminant transport problems in groundwater system. However, Fluidyn groundwater model-POLLUSOL is the most powerful software package which is based on the finite volume modelling hydraulic and concentration distribution. The other software tools like post processing particle tracking model (MODPATH), the finite difference groundwater model (FEMWATER), two-dimensional finite element model (SPEED 2D), multi-phase transport model (UTCHEM), transport tools and the transition probability geostatic software (T-PROGS) are also in use to model the contamination transport in groundwater and to predict the interaction between water bodies like surface and groundwater. The fluidyn POLLUSOL software tool is used specifically for simulating groundwater flows and predicting pollutant dispersion from a source through a porous medium. Flow equations are derived using conservation of total fluid mass principle and Darcy’s law for flow through porous media. Solute transport equation is derived from conservation of mass for a single solute or multiple solute species that may decay or adsorb in the porous medium.
Soil and water contamination are the most common problem encountered at the landfill sites in India. The objective of this study was to assess the possibility of reclamation of a closed landfill site located at Mavallipura near Bangalore, India. The existing liner system at this site has not completely prevented the leachate migration to the underlying aquifers, which is required to be managed and controlled to avoid adverse impact on the surrounding environment.
The soil profile, physicochemical parameters, hydraulic head and concentration levels of different contaminants were studied at this site. The batch leaching tests were conducted to know the heavy metal concentrations in the contaminated soil. Column tests were also conducted to estimate the migration rates of different contaminants through the soil. These migration rates were determined by the analytical solution of advection–dispersion equation using MATLAB software tool. The transport rates obtained from column tests along with the field data collected at the landfill site were given as input to the fluidyn-POLLUSOL model to simulate and assess the leachate migration from the landfill. This model may be useful to analyze the leachate migration from the landfill and its associated environmental impacts, particularly on groundwater wells and surface water ponds down gradient of the site. This research study is also useful to characterize landfill leachate through physicochemical analysis. Finally, this study also aims to serve as a guideline for the implementation of an appropriate treatments/remedial measures for reducing the adverse effects on the environment.
Materials and methods
The Mavallipura landfill site is in the north of Bangalore, India at Latitude 13°50′ North, Longitude 77°36′ East in the state of Karnataka. This landfill site has been used as a processing site for the municipal solid waste generated from the Bangalore city. Bangalore receives an average annual rainfall of 978 mm on long term basis. The primary rainy season is from June to September and the secondary rainy season is from November to December. The Mavallipura village is located about 20 km away from Bangalore. About 100 acres of land in and around the village is used for dumping Bangalore’s municipal waste by the Bruhat Bangalore Mahanagara Palike (BBMP–Greater Bangalore Municipal Corporation) that began accepting waste from 2005. The landfill was operated by M/s. Ramky Environmental Engineers from 2007 onwards and can sustain about 600 tonnes of waste per day. However, the BBMP has been sending almost 1000 tonnes of garbage from Bangalore city every day. Citizens around Mavallipura village have demanded to stop the landfill activities as it is illegal and unscientifically managed, and thus it is now closed for land filling. A soil liner of 0.3 m thickness has been applied historically, and the MSW is deposited in an unscientific manner that has resulted in steep, unstable slopes, leachate accumulation within the MSW mass, and leachate runoff into nearby water bodies such as ponds and opened wells.
The landfill site is about 100 acres out of which approximately 35 acres of land was used for landfill. The landfill has 3 cells at present. Cell 1 is filled and covered by a HDPE membrane. Cell 1 is spread over around 5 acres. Cells 2 and 3 are operational at present (Fig. 1 ). A single liner system was used in the landfill. The leachate collection systems are located outside the landfill. The MSW was dumped in the landfill in layers of 1–3 m. Waste pickers had access to recyclable materials before solid waste arrived at the landfill site. There are leachate collection sumps for the collection of the leachate. High Density Polyethylene (HDPE) pipes were used for the collection of the leachate. It is laid at a slope of 1:2. These pipes were connected to the leachate collection sumps.
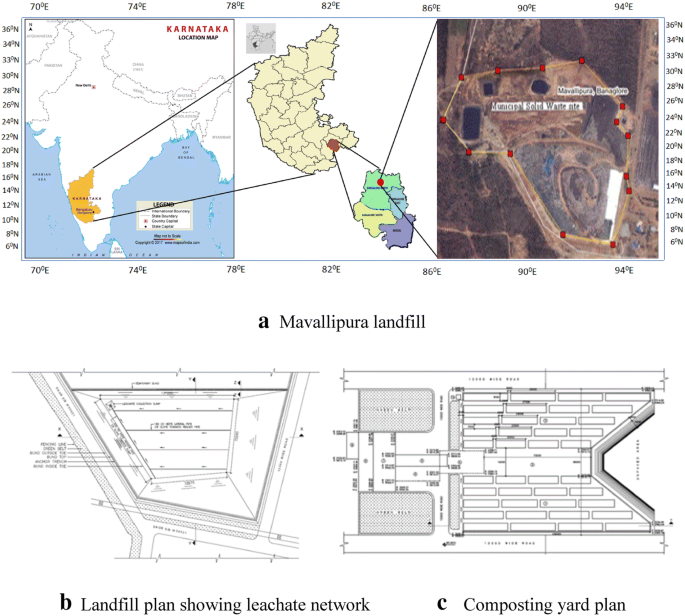
Mavallipura details
The high-density polyethylene geomembrane was made of high-density polyethylene resin which was extruded to extrusion sheet. The use of HDPE in landfill applications has set new standards for performance, durability, ease of installation and value. The specifications of the HDPE geomembrane are presented in Table 1 .
Landfill problems
In Mavallipura landfill, simply waste has been piling up in huge pits, around 40 m deep and spread over a few hectares. Since 2007, the landfill was receiving around 1000 tonnes daily MSW from the city (approximately a total of four million tonnes have been dumped at the landfill site so far). The unprocessed waste piled high in the pits resembles a large hillock (Fig. 2 ). This waste was brought from Bangalore city and dumped by the municipality and contractor’s trucks. This waste include newspaper, junk mail, food waste, raked leaves, dust grass clippings, broken furniture, abandoned materials, sewage sludge, industrial refuse, street sweepings, etc. The main issue arising in the landfill was the discharge of leachate which was formed by percolation of rain water through the waste and thus becoming contaminated with various biodegradable and nonbiodegradable pollutants. The subsequent movement of the leachate into the surrounding soil, surface water or groundwater resulted in severe pollution problems.
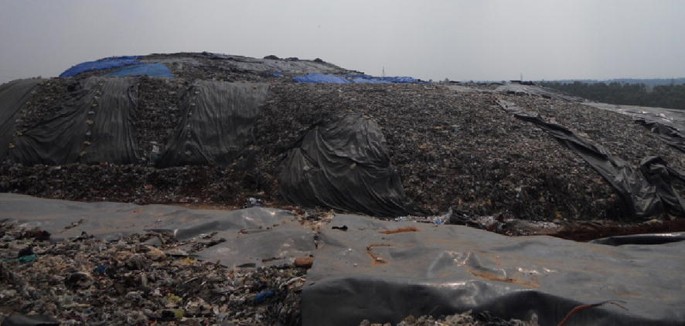
Waste covered with HDPE geomembrane sheets in landfill site
Sampling and physicochemical analysis
Figure 3 a shows a close view of sample locations marked on Google earth map. Figure 3 b shows the close view of sample locations at Mavallipura landfill site. A total of 3 samples one from landfill leachate and two from nearby surface water bodies were collected.
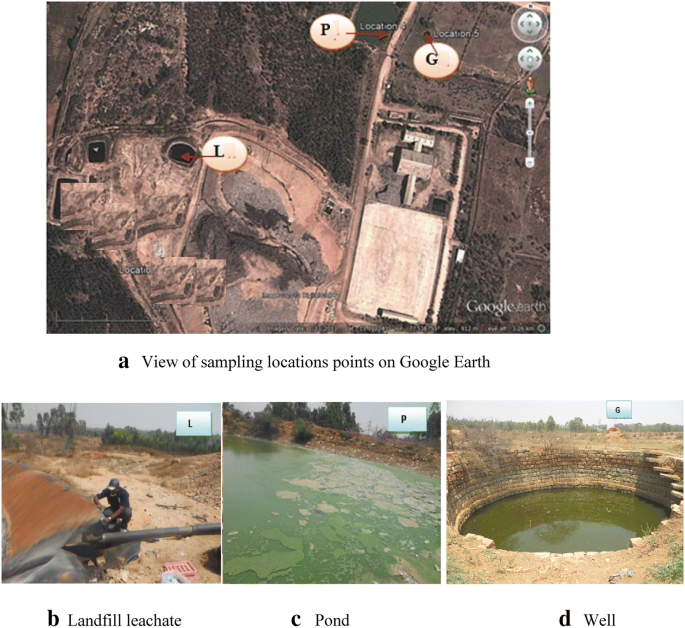
The site map and the sampling locations across leachate pond, storage and groundwater sources
In order to observe the spatio-temporal variations of the geochemistry of leachates and ground waters, one undiluted representative leachate sample (L, leachate collected directly from landfill) and another two samples of water from the nearby pond (P) and open well (G) were collected from downstream of Mavallipura landfill site in the month of April 2012. After the sample collections, this landfill site was abandoned and was restricted for any further treatment and disposal due to local agitation, therefore further sampling was not possible, and the analysis was carried out only for one season samples. The pH and electrical conductivity (EC) were recorded on site at the time of sampling with digital pH meter and digital EC meter, respectively. For the analysis of biological oxygen demand (BOD), samples were collected in 300 ml capacity BOD bottles. For heavy metal analyses, samples were separately collected in pre-washed polyethylene containers of 100 ml capacity and acidified (few drops of concentrated nitric acid were added to the leachate sample) onsite to avoid precipitation of metals. The samples were then transported in cooler boxes at temperature below 5 °C and transported immediately to the laboratory. The leachate samples were stored in refrigerator at 4 °C before proceeding for the analysis and during laboratory analysis, various ionic parameters were analysed. The analysis was carried out according to standard methods for examination of water and wastewater [ 18 ]. Table 2 shows the methods of analysis used for different parameters of leachate.
Contaminated soil collection
A contaminated soil was collected from an open dump located at the Mavallipura dumping yard, Bangalore. The samples were collected for every 1-m interval (0–1, 1–2 and 2–3 m) up to the full depth of the dump of about 3 m and the sampling was done with a 150-mm diameter manual auger. Approximately 100 kg of soil sample was collected from each location. For comparison purposes, bulk sampling at selected locations was carried out using JCB excavator machines and about 100 kg was collected by quartering method. Sample temperatures were measured immediately with a thermometer. Each sample was bagged in double plastic bags and labelled. All samples were transported to the laboratory where pH (of 1:10 water extract) and moisture content (at 105 °C) were determined. Then the samples were air dried by spreading on polythene sheets. The dried samples were screened into > 20 mm, 20–2 mm and < 2 mm fractions with a mechanical vibrating screen. The first two fractions were further segregated manually into individual constituents. The soil fraction (< 2 mm) was analysed for density, Volatile Organic Matter (VOM) at 550 ºC, and ash content. Total organic carbon (TOC) of these samples was determined using a solid model TOC Analyzer (Micro C, Analytic Jena. Germany). The composition of the soil is 29% clay, 19% silt and 52% sand. The soil type as per Indian Soil Classification System was observed as clayey sand (SC). The index properties of this soil are given in Table 3 .
Column test
For assessing the contaminant transport parameters of metal ions through soil, the column tests were conducted according to the specifications given in the literature [ 4 , 9 , 19 ]. The constant source concentration test condition was used to conduct the column experiments. The apparatus was designed to simulate the boundary conditions of a typical landfill liner system which is shown in Fig. 4 . The apparatus consists of an overhead tank mounted on a stand fixed to the wall and the soil column is connected to the overhead tank through a plastic pipe to allow seepage of solution through soil sample. Initial conditions were set as the moisture content and density of test samples as those during compaction. The constant source concentration for the influent solution was taken 100 ppm as the metal concentrations of selected chemical species were less than 100 ppm both in soil and leachate. The diameter and height of the columns were 4 cm and 10 cm, respectively. The hydraulic gradient adopted for the test was 10. The soil sample was placed in the column and the overhead tank was filled with water. The soil sample was completely saturated and then the permeability of the soil sample was measured. When steady flow conditions were established the simulated leachate was placed in the overhead tank and could pass through the soil sample. The source concentration was maintained constant throughout the test by adding same concentration of solution in the overhead tank and to maintain a constant head. The effluent samples were collected at regular time intervals till the required effluent concentration was obtained. The effluent volume was monitored at regular time intervals and concentrations were determined using Atomic Absorption Spectrophotometer (AAS).
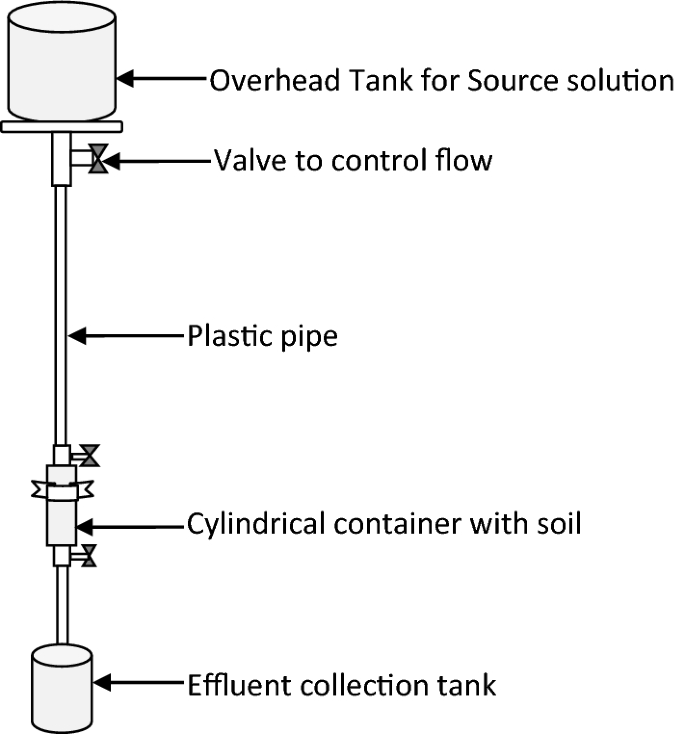
Schematic diagram of Column test apparatus
Batch leach test
Batch leaching tests were conducted on the contaminated soil (according to ASTM D3987) to know the initial concentrations of different contaminants present in the soil. An amount of 20 g of dry soil sample was mixed with 100 ml of distilled water. The mixture was shaken by a laboratory shaker for about 24 h. The solution was then centrifuged, and supernatant solution was collected. The supernatant solution was then filtered through a 0.45-μm pore size membrane filter. The concentrations of metals presented in the effluent solution were then measured by AAS and their quantities in the soil were estimated.
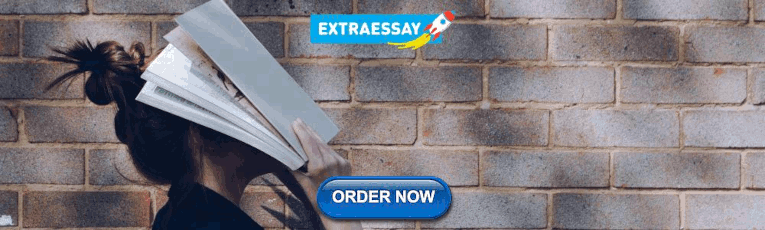
Advection–dispersion–reactive equation
The mass of conservative contaminant is transported by advection and dispersion processes. The governing linear partial differential equation for one-dimensional linear advection–dispersion–reactive process in vertical direction z for the unsteady state contaminant transport in a saturated homogeneous porous medium with uniform velocity field is given as:
where R is the dimensionless retardation factor defined as the ratio of the mean velocity of non-adsorbed solute to that of the retarded solute, c is the concentration of contaminant in the medium ( \({\text{M}}/{\text{L}}^{3}\) ), \(\partial {\text{c}}/\partial {\text{t}}\) is the change in concentration of contaminant with time \(( {\text{M}}/{\text{L}}^{3} /{\text{T)}}\) , \({\text{D}}_{\text{z}}\) is the longitudinal dispersion coefficient in z direction which takes into account both the molecular diffusion and the mechanical dispersion and is along the direction of flow and considered constant in this analysis, \(\partial {\text{c}}/\partial {\text{z}}\) is the change in concentration of contaminant with depth i.e. concentration gradient ( \({\text{M}}/{\text{L}}^{3} /{\text{L}}\) ), and \(\overline{\text{v}}_{\text{z}}\) is the average linear velocity in direction z which was considered steady and uniform in time and space. In Eq. ( 1 ) the term on the left side of the equation represents the change in concentration with time whereas the first term on the right-hand side accounts for the dispersion process and the second term for the advection process. In this formulation, the adsorption process induces retardation of the contaminant transport and so the advance of contaminant front is reduced as a result of sorption of the contaminant mass from solute to solid part of the porous medium.
Estimation of contaminant transport parameters
For the continuous point source of the contaminants, leaching across the soil surface boundary for infinite duration, Nelson [ 20 ] developed the analytical solution of Eq. ( 1 ) which gives the spatial and temporal distribution of concentration of contaminant. They used initial condition of c (z, t = 0) = 0 for z ≥ 0; and the Diriclet boundary conditions of c (z = 0, t) = \({\text{c}}_{\text{o}}\) for t ≥ 0 and c (z = \(\infty\) , t) = 0 for t ≥ 0 and solution was obtained as:
where \({\text{c}}_{0}\) is the concentration of the contaminant at the soil surface i.e. upstream boundary. The last boundary condition can only be appreciated mathematically. However, this is required to arrive at the analytical solution (Eq. 2 ). The term erfc is the complementary error function which is equal to 1 − erf. For estimation of contaminant transport parameters, the method as reported by Rowe et al. [ 19 ] was used. A computer program was prepared for the above equation using MATLAB v7 software tool to perform the iterations. The experimental values of the seepage velocity ( \(\overline{\text{v}}_{\text{z}}\) ), thickness of the soil sample (z), time periods (t) and the effluent concentrations along with the trial values of dispersion coefficient and retardation factor (R) were incorporated into the computer program. By running this program, the theoretical effluent concentrations were determined, and the plot was generated with the theoretical breakthrough curve. The theoretical effluent concentrations were compared with experimental values and the iterations were continued till the theoretical curve matched with the experimental values.
Results and discussions
Physico-chemical parameter analysis.
The important factors which influences the leachate quality such as municipal solid waste composition, elapsed time, temperature, moisture and available oxygen. Generally, the leachate quality with similar waste types may be different in different landfills located in varied climatic regions. Furthermore, operational practices in landfills also influence the leachate quality. The results of physicochemical characteristics of the leachates and water bodies from Mavallipura landfill are presented in Table 4 .
The pH value of leachate sample of the landfill site was observed to be 7.4. The pH values of the pond and well water samples were found to be 8.4 and 7.5, respectively. These variations were likely caused by several factors, such as rain water infiltration and dilution effects. Additionally, the influx of contaminants from natural and anthropogenic activities like percolation of solid waste leachates and other land uses can also affect the pH values. However, these pH values were within what would be considered a relatively normal band [ 21 ].
The Mavallipura leachate sample was found to have significantly high alkalinity values. The high alkalinity observed reflects the level of biodegradation process taking place within the disposal site. The presence of significant amounts of ash and slag in Mavallipura landfill site is from the combustion of wood, agricultural residues and peat. These components are known to increase alkalinity greatly in leachates. The high concentration founds to be high in water samples this could be due to the reduced solubility of heavy metals and have effect on health. Also, this leads to unpleasant odour in the water sample and is unacceptable for many users. The high alkalinity values observed, therefore, imply that the groundwater is contaminated.
Mavallipura landfill leachate sample was found to have considerably high concentrations of all the major anions like chlorides, nitrates, sulphates as comparison with these in samples from other sites. The concentration of chloride highest, while sulphate concentration was the lowest. The high chloride content in the leachate sample reflects the significant presence of soluble salts in the municipal solid waste materials of the study area. The high chloride content in Mavallipura landfill leachate sample is attributed to the large amount of sewage, agricultural and another animal waste deposited in the site. Prior to anaerobic activity sulphate is converted to sulphide and metal sulphide precipitates in leachate sample.
Sulphates were found to be high in the leachate sample due to the decomposition of organic matter, soluble waste, such as construction wastes or ash, synthetic detergents and inert waste, such dredged river sediments. Similarly, nitrates represent the most oxidized form of nitrogen found in natural system. It is often regarded as an unambiguous indicator of domestic and agricultural pollution. In leachate sample it was formed primarily as a result of oxidation of ammonium to nitrite and subsequently, to nitrates by nitrification process.
The constituent’s calcium, magnesium, sodium and potassium are considered generally to be major cations typically present in leachate. In leachates, these are sourced usually from the degradation of organic materials and the dissolution of inorganic wastes such as concrete, plaster and tiles. Sodium and potassium are both present at considerably high concentrations in the entire samples of this investigation. The sodium and potassium are not affected significant by microbiological activities within the landfill site.
Calcium is one of the most common cations found in ground water aquifers, as it dissolves from rocks, such as limestone, marble, calcite, dolomite, gypsum, fluorite and apatite. In addition, with magnesium, it is one of the principal cations associated with water hardness. Calcium concentrations were noticeably high in well sample.
Concentrations of heavy metals were found to be low in leachates samples except for Fe and Zn. Heavy metals appear in the landfill from sources like batteries, consumer electronics, ceramics, light bulbs and glass can all introduce metal contaminants into the solid waste stream. Concentration of heavy metals in a landfill is generally higher at earlier stages because of higher metal solubility as a result of low pH caused by production of organic acids. It is now recognized that most trace elements are readily fixed and accumulate in soil sand because this process is largely irreversible, repeated applications of amounts in excess of plant needs eventually contaminate the soil and may either render it non-productive or the product unusable. Although plants do take up the trace elements, but the uptake is normally so small that this alone cannot be expected to reduce appreciably the trace element.
The quality assurance mainly depends on the accuracy and reliability of the information used. This is very necessary for water quality assessments in which the compositional characteristics of a sample can change rapidly when influenced by environmental factors. Hence, it is important to process the sampling and analyse to achieve high-quality analytical results. It is well documented that within water bodies samples, the number of positively cations should balance the number of negatively charged anions. Cation–anion balance is calculated by comparing the total charge of the positive-charged ions (cations) with the total charge of the negative-charged ions (anions). Ionic balance error for ground water samples should not exceed 5% unless where the TDS value is less than 5 mg/l, in which case a higher error is tolerable (UNEP/WHO, [ 22 ]). The ion compositions were plotted for all water bodies samples with an error of ion balance within the ± 5% range. Major cations such as Na + , K + , Ca 2+ and Mg 2+ and major anions such as HCO 3− , CO 3 2− , SO 4 2− and Cl − were plotted in a hydrochemical trilinear diagram, also known as a Piper diagram. Figure 5 shows the Piper diagram, composition of leachate samples (L) according to their major cations and anions. The ionic balances were calculated and examined for each sample. The major anions considered were total alkalinity, chlorides, sulphates and nitrates, while the major cations included are calcium, magnesium, sodium and potassium. Leachate sample whose error of ions was within ± 5% were plotted.
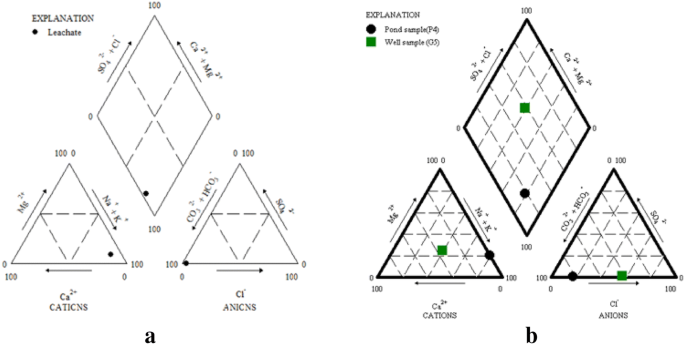
Piper diagram, composition of a leachate samples; b water bodies samples
The piper diagram reveals composition of different ions in percentage. A major conclusion can be drawn from the piper diagram of the collected leachate samples. More importantly it indicates predominance of select cations as Na + and K + in comparison to calcium and magnesium. Bicarbonates and carbonates are the dominant anion found in the leachate samples compared to sulphates and chlorides. High chloride builds up are possibly due to leaching of percolating water from the MSW. The analyzed leachate sample can be thus categorized as the Na–HCO 3 .
Also, piper diagram identifies the hydrogeochemical facies. Based on piper diagram a few conclusions can be inferred of the collected water’s bodies samples. Firstly, it indicates large percentages of the samples within the Ca–SO 4 category followed by Na–HCO 3 type. Secondly, bicarbonates and carbonates dominated the anions compared to chloride. However, anions like sulphates were very meager in concentrations in relation to other anions. Chloride content is high in ground water can be possibly domestic effluents, fertilizers, septic tanks, and leachate. Moreover, the above plot shows Ca 2+ concentrations being noticeably high in well sample. However, Na + and K + dominated the pond samples.
Contamination transport model
Once the landfill leachate is released into the subsurface, contaminants will interact physically, hydrologically and biochemically with both the water bodies and the soil matrix. A complete transport model must therefore account for multispecies physical and biochemical transport processes. Fluidyn groundwater model-POLLUSOL is the most powerful software package designed specifically for continuous simulating 3-dimensional groundwater flows and predicting pollutant dispersion from a source through porous medium. Flow equations for flow into porous medium are employed using conservation of total fluid mass principle and Darcy’s law. Solute transport equation is derived from conservation of mass for a single solute or multiple solute species that may decay or adsorb in the porous medium. Simulation was carried out simultaneously for groundwater flow and pollutant dispersion using the built-in solver of fluidyn -POLLUSOL which is based on the finite volume method. The simulations were carried out in steady state mode. The generalized Navier–Stokes equations, for porous media, were solved to obtain the groundwater flow field. The contaminant transport parameters of the species of interest in the porous media were determined through column tests using the procedure as described in “ Contaminated soil collection ” and “ Column test ” sections. These parameters are given as input data for generating output from the fluidyn -POLLUSOL model. The contaminant transport parameters determined by matching the theoretical breakthrough curve with the experimental results are shown in Figs. 6 , 7 and 8 . The contaminant transport parameters of the test samples along with their physical properties are as given in the Table 5 . These parameters are given as input data for generating output from the fluidyn-POLLUSOL model.
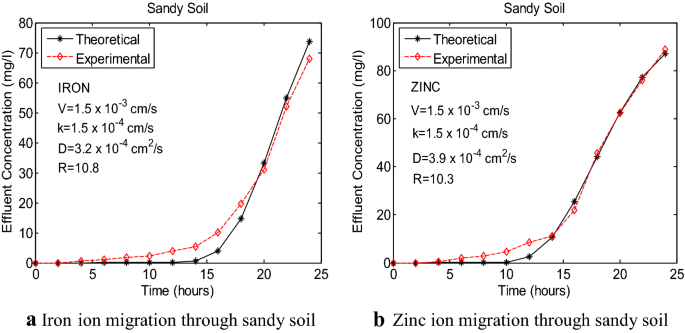
Migration of ions through sandy soil
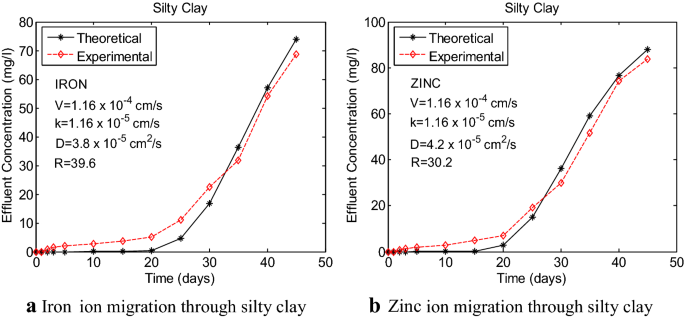
Migration of ions through silty clay
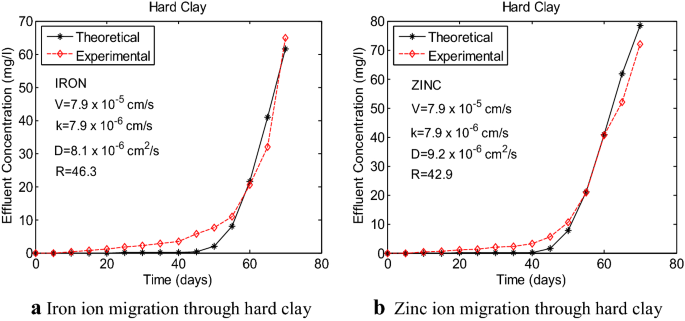
Migration of ions through hard clay
It can be observed that the dispersion coefficients of hard clay are much lower and distribution coefficients are much higher than those of silty clay and sandy soil even though the density of hard clay sample is less than the other samples. This effect is due to the presence of more clay fraction presented in the hard clay which causes less hydraulic conductivity and more adsorption capacity. From the test results (Figs. 6 , 7 , 8 ), it is evident that the dispersion coefficients of Iron are less than that of Zinc whereas the retardation factors of Iron are higher than Zinc in all the three soils studied. This may be due to the less mobility of Iron caused by higher specific gravity of Iron compared to Zinc. The specific gravity of Iron varies from 7.6 to 7.9 whereas it varies from 6.9 to 7.2 for Zinc. This affinity order of Cu and Zn (Cu > Zn) for soils studied is similar to that obtained in the previous studies of Matos et al. [ 23 ], Young et al. [ 24 ], Soares [ 25 ], Vega [ 26 ], Nascentes et al. [ 12 ] and Korf et al. [ 9 ]. However, the differences in the transport parameters of iron and Zinc are relatively very less in the soils studied.
Fluidyn’s groundwater model
The contour data of the terrain (topo map) of Mavallipura landfill, is used to generate the computational domain. The borehole data, at the landfill site, was used for modelling the entire domain. According to the borehole data there are three layers of soil at the location, namely sandy, silty clay and hard clay occurring in the same order. Sandy soil was at the top and hard clay was at the bottom next to the impervious bed rock as shown in Fig. 9 .
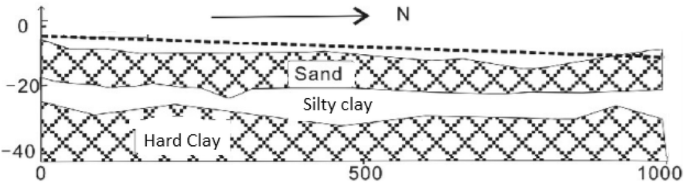
Conceptual cross-section of the modeled area
The building cover is considered by making the surface, on which the building stands, impervious to the rainfall. The computational domain covers an area of 0.372 sq. km and a soil depth of 6 m. The geometrical model of the terrain and the leak detected are shown in Fig. 10 a. The leachate can seep into the soil at a location next to the landfill. Initially, the location of the leak is selected by assuming. The area of the leak is 31.5 m 2 (approx.). The volume flow rate of the leak is arbitrarily chosen to be 10 ml/s. The selection of the pollutants to be modeled was based on the corresponding concentrations in site specific leachate samples, susceptibility to natural attenuation and drinking water standards. The physicochemical analysis revealed that iron (Fe) and zinc (Zn) would be the most critical indicators. So, concentration of the pollutants being considered (Fe and Zn), in the leachate at the leak, was set to 1. Also, the simulation was done for a worst-case scenario.
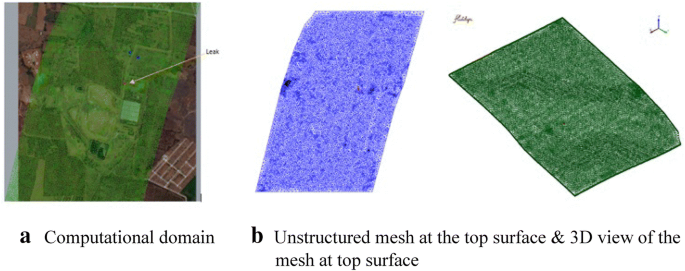
Finite volume mesh
Rainfall data was collected from the Indian Meteorological Department. The average annual rainfall in Bangalore is 978 mm. This data has been used to provide constant and uniform rainfall over the computational domain, for the first simulation. The average monthly rainfall during monsoon is ~ 171 mm. This data has been used to provide constant and uniform rainfall over the computational domain, for the second simulation. A nearly uniform unstructured mesh was generated for the study. The mesh created is made of layered unstructured cells (prismatic). The mesh used contains 333,536 cells (Fig. 10 b).
The groundwater velocity vector plots in the sandy soil layer, for average rainfall and monsoon conditions, are shown in the Fig. 11 . The velocity vectors in the top half of the silty clay layer is like the ones in the sand layer but lesser in magnitude by about one order. The velocity vectors in the lower half of the silty clay layer and the hard clay layer are almost nonexistent. The blue dots in the Figs. 12 , 13 , 14 , 15 , 16 , 17 , 18 and 19 indicate the locations of the water bodies.
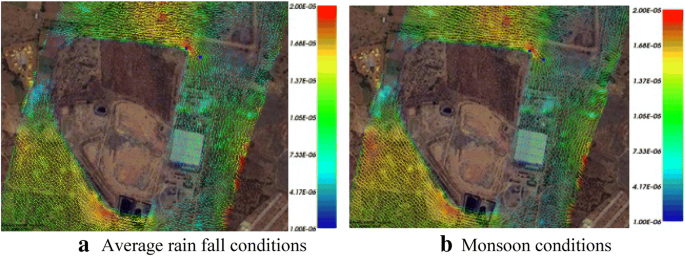
Groundwater velocity vector plots in the sandy soil layer
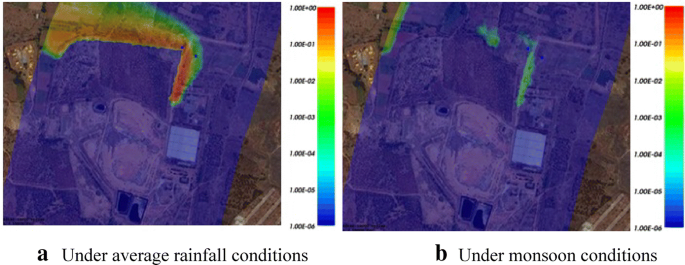
Normalized concentration of zinc in the sandy layer
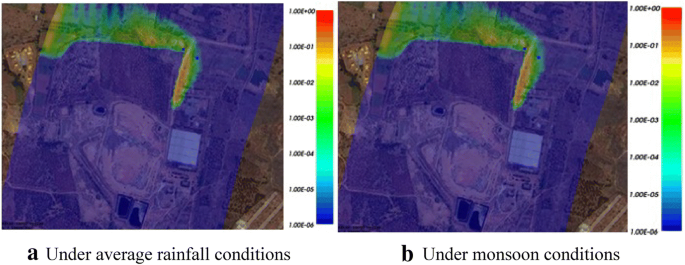
Normalized concentration of zinc in the top half of silty clay layer
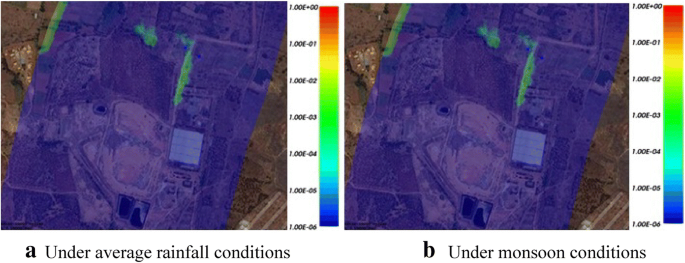
Normalized concentration of zinc in the bottom half of silty clay layer
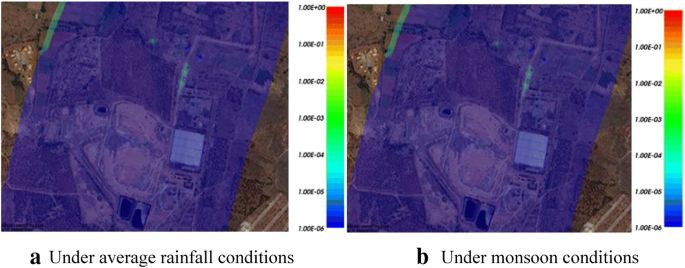
Normalized concentration of zinc in the hard clay layer
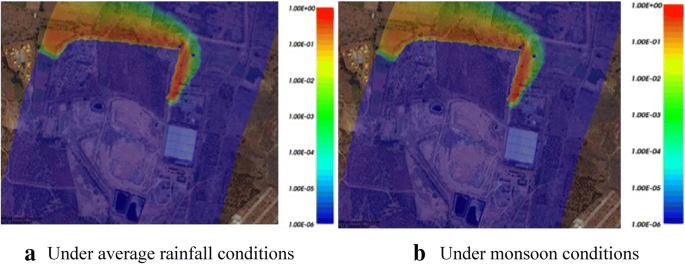
Normalized concentration of iron in the sandy layer
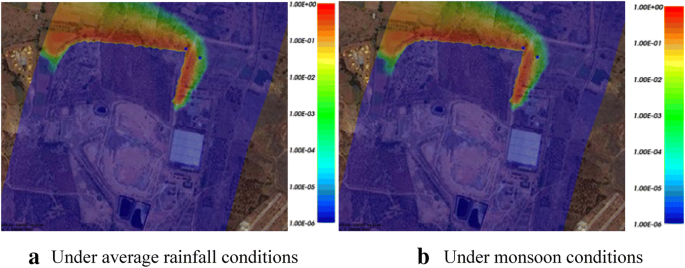
Normalized concentration of iron in the top half of the silty clay layer
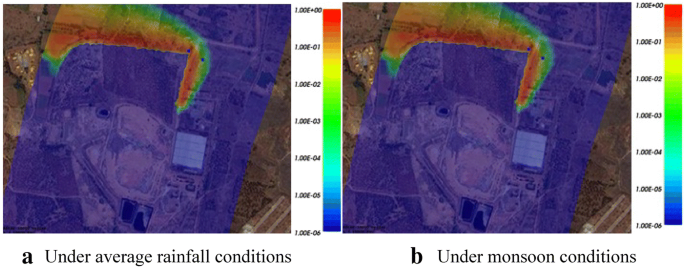
Normalized concentration of iron in the bottom half of the silty clay layer
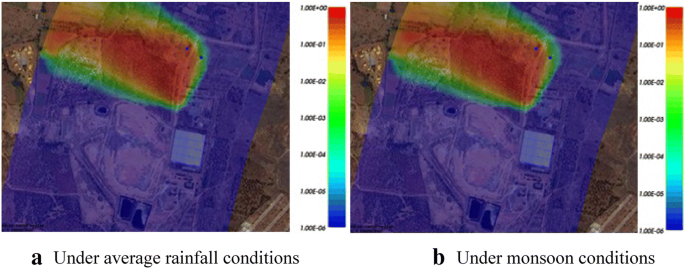
Normalized concentration of iron in the hard clay layer
As shown in Fig. 9 , silty clay is the thin layer. The thin horizontal layer of silty clay extends across the flow domain (Figs. 13 , 14 , 15 ), it causes the contaminants to move through the flow system almost entirely in this thin layer. The total travel time would be one-fifth and sandy layer of higher conductivity has a 100-fold larger value of k than the rest of the system and exerts a very strong influences on the migration patterns and velocity distribution.
The velocity vectors clearly show how the pollutants from the leak reach the water bodies. While for the pond (the blue dot towards north-west), the pollutant reaches merely by means of advection, for the well (the blue dot towards southeast), its pollution would depend also on the diffusion. This result justifies positioning the leak just after the building cover such that crosswind diffusion is free to take place and achieve maximum dispersion. The distribution of zinc and iron in the various layers, under average rainfall and monsoon conditions, is shown in the Figs. 12 , 13 , 14 , 15 , 16 , 17 , 18 and 19 . The plots for average rainfall and monsoon conditions do not show much difference. However, the rainfall may have a linear effect only.
Several forces react with the leachate migration resulting in changes in chemistry and reduction of strength from the original release. The physical forces like filtration, sorption, advection and dispersion and chemical forces such as oxidation–reduction, precipitation–dissolution, adsorption–desorption, hydrolysis and ion exchange, and biological reaction like microbial degradation. These reactions will depend on the soil stratification of the landfill, the hydraulic characteristics of the ground water system, and the chemistry of the leachate. Also, these reactions have the capability to reduce the potential impact to groundwater and surface water.
Mavallipura landfills are constructed with liners that contain leachate, and leachate collection systems that collect it. But due to unsegregated and mismanagement of landfill, leads to puncture the linear system in the landfill. This resulted in creating significant leachate-contaminated to the pond water and openwell water environment, it will migrate downward through the unsaturated zone until it reaches the saturated zone. Finally, leachate then will follow the hydraulic gradient of the groundwater system.
Conclusions
Results of physicochemical parameters and determined the contents of heavy metals leaching from Mavallipura landfill suggest an exceptional pollution. High contents of organic pollutants and heavy metals found on landfill sites, as well as the concentration of these metals in ponds and open wells in Mavallipura. Iron and zinc concentrations are higher in leachate sample.
Physicochemical analysis reveals that there is high contamination of organic & inorganic constituents. Heavy metals concentration was in trace only indicating that the waste dumped is predominantly municipal waste. Based on physicochemical analysis the quality of water was found to be unsuitable for drinking purpose.
The study revealed that the concentration of MSW materials in the landfill site had systematically polluted the soil and water bodies (surface water and groundwater) over time. The effect of such pollution as assessed from the study declined away from the polluting source. This implied that the surface and groundwater bodies were more dependent on proximity to landfill sites. This dependence may be due to the influence of topography, type, state of waste disposal system and to some extent, the hydrogeology of the site.
Based on the groundwater model, the results revealed that the zinc concentration observed was being limited to upper layers of the soil, owing to the large time required in seeping through the layers and its radioactively decaying tendency. At the same time, the iron concentration showed the maximum dispersion that could be achieved by any non-decaying pollutant. The results make it evident that under the assumed conditions, it is highly probable that the groundwater flow could cause contamination of the water bodies in the vicinity of the landfill due to a leak at the landfill. This leak area has to be repaired either by laying a new liner or by using some cement grouts to close the leak. Constructing a new liner for the leak area may be difficult as it is required to excavate the landfill and hence grouting is a better option to close the leak area with cementing grouts. As the water bodies surrounding the landfill are already contaminated, it is recommended to treat them using pump and treat technique.
Abbreviations
dimensionless retardation factor
concentration of contaminant
change in concentration of contaminant
average linear velocity
time period
dispersion coefficient
Gzar HA, Abdul-Hameed A, Yahya AY (2014) Extraction of lead, cadmium and nickel from contaminated soil using acetic acid, open. J Soil Sci 4:207–214
Google Scholar
Mulligan CN, Yong RN, Gibbs BF (2001) Remediation technologies for metal-contaminated soils and groundwater: an evaluation. Eng Geol 60:193–207
Article Google Scholar
Jaishankar M, Tseten T, Anbalagan N, Mathew BB, Beeregowda KN (2014) Toxicity, mechanism and health effects of some heavy metals. Interdiscipl Toxicol 7(2):60–72
Almani ZA, Pathan AF, Memon AA (2013) Heavy metal diffusion through soft clay under high hydraulic gradients. Mehran Univ Res J Eng Technol 32(2):307–318
Azambuja E, Cancelier DB, Nanni AS (2000) Soil contamination by LNAPL: diagnosis and remediation discussion, in Portuguese, GEOSUL 2000. http://www.azambuja.com.br/acervo/geosul2000.pdf . Accessed 18 June 2006
Azevedo ICD, Nascentes RC, Matos TA, Azevedo FR (2005) Transport parameters determination of heavy metals in an oxisol compacted. Revista de Engen- haria Agrícola e Ambiental 9(4):623–630 (in Portuguese)
Delgado AWC (2002) Transport mechanisms of heavy metals, national environmental congress 2. Mini Course, Salvador (in Portuguese)
Knop A (2007) Study the behavior of liners attacked by sulfuric acid, Ph.D. Thesis, Universidade Federal do Rio Grande do Sul, Porto Alegre (in Portuguese)
Korf EP, Thomé A, Consoli NC, de Souza Tímbola R, dos Santos GC (2011) Metal transport parameters in residual soil with an undisturbed and remolded structure percolated by an acid solution. J Environ Prot 2(8):1076
Krahn J (2004) Transport modeling with CTRAN/W: an engineering methodology, CTRAN user’s manual, 1st edn. GEOSLOPE International Ltd., Calgary
Moncada MPH (2004). Laboratory Study of Collapse Characteristics and Solute Transport Associated with the Infiltration of Caustic Liquor in a Laterite Soil, Master’s Thesis, Departamento of Civil Engineering, Pontifícia Universidade Católica do Rio de Janeiro, Rio de Janeiro (in Portuguese)
Nascentes R., Ernani L. P., Silva P. S. and Azevedo I., (2007). Heavy Metals Removal with the Use of Coal, Proceedings VI REGEO, Recife (Brazil), 2007, (in Portuguese)
Ochola C, Moo-Young H (2004) Evaluation of metal attenuation through paper clay utilized for containment of contaminated groundwater. J Environ Eng 130(8):873–880. https://doi.org/10.1061/(ASCE)0733-9372(2004)130:8(873)
Shackelford CD (1993) Contaminant transport, geotechnical practice for waste disposal. Chapman & Hall, London. pp 33–65. https://doi.org/10.1007/978-1-4615-3070-1_3
Shackelford CD (1995) Cumulative mass approach for column test. J Geotechn Eng 121(10):696–703. https://doi.org/10.1061/(ASCE)0733-9410(1995)121:10(696)
Sharma HD, Reddy KR (2004) Geoenvironmental engineering: site remediation, waste containment, and emerging waste management technologies. Wiley, New Jersey, p 992
Yong RN, Mohamed AMO, Warkentin BP (1992) Principles of contaminant transport in soils. Elsevier, Amsterdam, p 327
APHA (1998) Standard methods for examination of water and waste water, 19th edition, American Public Health Association, Washington, DC. Brock TD, Madigan MT, eds. Biology of Microorganisms. 5th edn. Prentice Hall International Inc., New Jersey. pp 641–644
Rowe RK, Chris J, Caers CJ, Barone F (1988) Laboratory determination of diffusion and distribution coefficients of contaminants using undisturbed clayey soil. Can Geotech J 25(1):108–118
Nelson RW (1966) Flow in heterogeneous porous mediums: 1. Darcian-type description of two-phase systems. Water Resour Res 2(3):487–495
Naveen BP, Mahapatra DM, Sitharam TG, Sivapullaiah PV (2017) Ramachandra TV (2017) Physico-chemical and biological characterization of urban municipal landfill leachate. Environ Poll. 220:1–12
WHO (2006) Protecting ground water for health: managing the quality of drinking-water sources. Schmoll O, Howard G, Chilton J, Chorous I. ISBN: 1843390795. IWA, London
Matos AT, Fontes MPF, Costa LM, Martinez MA (2001) Mobility of heavy metals as related to soil chemical and mineralogical characteristics of Brazilian soils. Environ Poll 111(3):429–435. https://doi.org/10.1016/S0269-7491(00)00088-9
Young RN, Yaacobl WZW, Bentley WSP, Harris C, Tan BK (2001) Partitioning of heavy metals on soil samples from column tests. Eng Geol 60:307–322
Soares MR (2004) Distribution coefficient (Kd) of heavy metals in soils of São Paulo, Ph.D. Thesis, Escola Superior de Agricultura Luiz de Queiroz, Universidade de São Paulo, Piracicaba (in Portuguese)
Vega FA (2006) Competitive sorption and desorption of heavy metals in mine soils: influence of mine soil characteristics. J Coll Interface Sci 298(2):582–592
Download references
Authors’ contribution
BPN entire manuscript written and detailed analysis and modelling is done. JS contribution as mentioned below: column test, batch test. RKM contribution as mentioned below: mathematical equation developed. All authors read and approved the final manuscript.
Acknowledgements
The authors would like to express their sincere gratitude to fluidyn-POLLUSOL for their suggestions and help.
Competing interests
The authors declare that they have no competing interests.
Publisher’s Note
Springer Nature remains neutral with regard to jurisdictional claims in published maps and institutional affiliations.
Author information
Authors and affiliations.
Amity University Gurgaon, Gurgaon, Haryana, India
B. P. Naveen & R. K. Malik
Ramaiah Institute of Technology, Bangalore, India
J. Sumalatha
You can also search for this author in PubMed Google Scholar
Corresponding author
Correspondence to B. P. Naveen .
Rights and permissions
Open Access This article is distributed under the terms of the Creative Commons Attribution 4.0 International License ( http://creativecommons.org/licenses/by/4.0/ ), which permits unrestricted use, distribution, and reproduction in any medium, provided you give appropriate credit to the original author(s) and the source, provide a link to the Creative Commons license, and indicate if changes were made.
Reprints and permissions
About this article
Naveen, B.P., Sumalatha, J. & Malik, R.K. A study on contamination of ground and surface water bodies by leachate leakage from a landfill in Bangalore, India. Geo-Engineering 9 , 27 (2018). https://doi.org/10.1186/s40703-018-0095-x
Download citation
Received : 01 September 2018
Accepted : 04 December 2018
Published : 12 December 2018
DOI : https://doi.org/10.1186/s40703-018-0095-x
Share this article
Anyone you share the following link with will be able to read this content:
Sorry, a shareable link is not currently available for this article.
Provided by the Springer Nature SharedIt content-sharing initiative
- Concentration
- Leachate contaminant
- Find a journal
- Publish with us
- Track your research
- Open access
- Published: 03 April 2024
Heavy metal association with chronic kidney disease of unknown cause in central India-results from a case-control study
- Mahendra Atlani 1 na1 ,
- Ashok Kumar 2 na1 ,
- Rajesh Ahirwar 3 ,
- M. N. Meenu 1 ,
- Sudhir K. Goel 2 ,
- Ravita Kumari 2 ,
- Athira Anirudhan 1 ,
- Saikrishna Vallamshetla 4 &
- G. Sai Tharun Reddy 4
BMC Nephrology volume 25 , Article number: 120 ( 2024 ) Cite this article
Metrics details
Chronic Kidney Disease of unknown cause (CKDu) a disease of exclusion, and remains unexplained in various parts of the world, including India. Previous studies have reported mixed findings about the role of heavy metals or agrochemicals in CKDu. These studies compared CKDu with healthy controls but lacked subjects with CKD as controls. The purpose of this study was to test the hypothesis whether heavy metals, i.e. Arsenic (As), Cadmium (Cd), Lead (Pb), and Chromium (Cr) are associated with CKDu, in central India.
The study was conducted in a case-control manner at a tertiary care hospital. CKDu cases ( n = 60) were compared with CKD ( n = 62) and healthy subjects ( n = 54). Blood and urine levels of As, Cd, Pb, and Cr were measured by Inductively Coupled Plasma- Optical Emission Spectrometry. Pesticide use, painkillers, smoking, and alcohol addiction were also evaluated. The median blood and urine metal levels were compared among the groups by the Kruskal-Wallis rank sum test.
CKDu had significantly higher pesticide and surface water usage as a source of drinking water. Blood As levels (median, IQR) were significantly higher in CKDu 91.97 (1.3–132.7) µg/L compared to CKD 4.5 (0.0–58.8) µg/L and healthy subjects 39.01 (4.8–67.4) µg/L ( p < 0.001) On multinominal regression age and sex adjusted blood As was independently associated with CKDu[ OR 1.013 (95%CI 1.003–1.024) P < .05].Blood and urinary Cd, Pb, and Cr were higher in CKD compared to CKDu ( p > .05). Urinary Cd, Pb and Cr were undetectable in healthy subjects and were significantly higher in CKDu and CKD compared to healthy subjects ( P = < 0.001). There was a significant correlation of Cd, Pb and Cr in blood and urine with each other in CKDu and CKD subjects as compared to healthy subjects. Surface water use also associated with CKDu [OR 3.178 (95%CI 1.029–9.818) p < .05).
The study showed an independent association of age and sex adjusted blood As with CKDu in this Indian cohort. Subjects with renal dysfunction (CKDu and CKD) were found to have significantly higher metal burden of Pb, Cd, As, and Cr as compared to healthy controls. CKDu subjects had significantly higher pesticide and surface water usage, which may be the source of differential As exposure in these subjects.
Peer Review reports
Chronic kidney disease of unknown cause (CKDu) has been reported in various parts of the world (i.e., Nicaragua, El Salvador, Sri Lanka), including India, as an endemic disease. The disease is a diagnosis of exclusion, made when a patient fulfils the Kidney Disease Improving Global Outcomes (KDIGO) CKD criteria but without the evidence of a recognized cause such as diabetes, hypertension, or glomerulonephritis [ 1 ]. No uniform and definite cause has yet been identified, though various environmental factors have been associated with and suggested to play a role in the pathogenesis. For example, heat stress, strenuous exercise, agrochemicals, and heavy metals have been held responsible for Mesoamerican nephropathy [ 2 ]. Mixed evidence has been reported for association with agrochemicals, heavy metals, and genetic variability for CKDu in Sri Lanka [ 3 , 4 , 5 ]. In India, one small study reported an association of heavy metals with CKD [ 6 ]. A study done on groundwater samples from the Uddanam region of Andhra Pradesh (India), which has a high prevalence of CKDu reported water as acidic (pH < 6.5) and to contain higher silica and lead in wet and dry seasons, respectively. Phthalates were also detected in the groundwater [ 7 ]. Previous studies have attempted to find a correlation between heavy metals and CKDu by comparing cases and endemic and nonendemic controls [ 4 ]. No study has yet tried to find the association of heavy metals comparing CKDu with CKD. Furthermore, previous studies have used urinary metal levels as a biomarker of metal exposure. There is an inherent issue of reverse causality i.e., reduced excretion of metals in urine with a reduction in glomerular filtration rate (GFR) [ 8 ]. Measurement of metals in blood has also been reported to be a promising biomarker of metal exposure [ 9 , 10 ]. Some studies have employed urine to blood ratio for deciding whether urine or blood levels should be used for a particular metal. For metals with urine/blood ratio more than one blood metal levels, whereas for metals with urine/blood ratio less than one, urine metal levels were used in estimation analysis [ 11 ]. The purpose of this study was to test hypothesis whether heavy metals i.e. Arsenic (As), Cadmium (Cd), Lead (Pb) and Chromium (Cr) are associated with CKDu, in central India using blood and urine levels as biomarker of metal exposure.
Materials and methods
Study setting and population.
Study was conducted in a tertiary care hospital setting in the Department of Nephrology in India in a case-control design between December 2019 to June 2022. Participants were enrolled between December 2019 -December 2021. The data collection was done simultaneously. The sample analysis was carried out between January to June-2022. The study was performed according to the guidelines of the Declaration of Helsinki. The study objective was to compare CKDu cases with CKD and healthy controls with regard to biomarkers of exposure of heavy metals [blood and urine levels of cadmium (Cd), lead (Pb), arsenic (As) and chromium (Cr)]. The study included adults aged 18–70 years with CKDu and two groups of the control population, one with CKD and another group of healthy controls without evidence of CKD.
The CKDu and CKD cases were inducted among the patients visiting the nephrology outpatient department and based on pre-defined criteria. At the same time, healthy controls were inducted among the healthy relatives accompanying the patients visiting other departments of the institute for treatment. Written informed consent was obtained from all the participants.
The case definition of CKDu was based on criteria proposed by the Indian Society of Nephrology for the diagnosis of CKDu [ 12 ]. The inclusion criteria included- eGFR < 60 mL/min/1.73m2 (CKD-EPI) [ 13 ] and albumin-to-creatinine ratio (ACR) > 30 mg/g for more than 3 months with:
Urine protein creatinine ratio(PCR) less than 2g/g.
No history of glomerulonephritis, pyelonephritis, renal calculi, polycystic kidneys or obstruction on renal ultrasound.
Not on treatment for diabetes and HbA1c less than 6.5%.
Blood pressure less than 140/90 if CKD stage 1 and 2; and less than 160/100 if CKD stage 3,4, and 5 and on a single drug for blood pressure control.
Case definition of CKD was based on: eGFR < 60 mL/min/1.73m2 (CKD-EPI) and albumin-to-creatinine ratio > 30 mg/g for more than 3 months. Patients were included in the CKD group only if PCR > 2g/g. Hypertension with BP > 140/90 in stages 1–2 and > 160/100 in stages 3–5 or on two or more drugs for BP control.
CKD staging was based on the KDIGO-2008 classification [ 1 ]. The same stages were applied to categorize the renal functions of subjects with CKDu.
Inclusion criteria for healthy controls included: Absence of CKD as evidenced by eGFR more than 90 ml/min/1.73m2, ACR < 30mg/g and lack of anatomical renal disease, obstruction or stone on renal ultrasound, no history of diabetes, HbA1C less than 6.5 and BP less than 140/90.
Biases were kept a minimum by adhering to the case definition described above, and study exposures are mainly objectively assessed with very less dependency on recall i.e. for pesticide or painkiller use. The urine metal levels were adjusted for urine dilution by estimating metals per gram of creatinine in urine.
Sample size
Assuming a difference of moderate effect size (0.25), between three groups (CKDu cases, CKD Controls, Normal Controls) with a confidence level of 95% and power of 80%, the calculated sample size was 159. The final sample size estimated, including a 10% non-response rate, was 180 (60 per group).
Specimen collection and analysis
For the analysis of heavy metals, venous blood (2 ml) was collected in trace element free Trace Element K2-EDTA Vacutainer (Cat# BD 368381). Whole blood was stored at -40 °C until analysis. Ten millilitres (10 ml) of first-morning urine was collected in 50 ml polypropylene tubes. Urine was stored at -40 °C in aliquots until analysis. Serum and urine creatinine was measured using a modified kinetic Jaffe’s method using a Random Access Fully Automated Chemistry Analyzer (Beckman Coulter). Urinary protein and urine albumin were estimated using a colorimetric and immune-turbidimetric methods, respectively, using a Random Access Fully Automated Chemistry Analyzer (Beckman Coulter). HbA1c was analyzed by ion-exchange high pressure liquid chromatography method using a D10 Haemoglobin testing system (BioRad Laboratories). eGFR was calculated from serum creatinine and CKD -EPI equation (Ref). A kidney ultrasound was performed in standard B Mode grey scale in 3.5–5 MHz, the longitudinal length was measured along with the width and thickness of the kidney, renal stones, and any other anatomical abnormality.
Estimation of heavy metals in blood and urine
Levels of Cd, Pb, Cr and As were measured in whole blood and urine. Urinary spot sample results of metal analysis were adjusted for dilution by urine creatinine. Metal analysis was carried out at NIREH, Bhopal (India).
Levels of various heavy metals, viz. Cd, Pb, Cr, As in the collected blood and urine samples were analyzed through inductively coupled plasma optical emission spectroscopy (iCAP® 7400 Duo ICP-OES, ThermoFisher Scientific® Pvt. Ltd). Blood and urine samples were acid-digested in a microwave oven prior to metal detection on ICP-OES. For blood digestion, 1 mL of whole blood sample was mixed with 6 ml of a freshly prepared mixture of concentrated trace metal grade nitric acid (HNO3) and hydrogen peroxide (H2O2) in a ratio 2:1 (v/v) in high-purity polytetrafluoroethylene (PTFE-TFM) vessels. For urine digestion, 5 mL of urine sample was mixed with 6 ml of a freshly prepared mixture of HNO3 and H2O2 in a ratio of 2:1. After gentle mixing of these reactants with blood, the PTFE-TFM vessels were arranged in the rotor (24HVT80, Anton PAAR) and digestion was carried out in the Anton Paar, multi microwave PRO Reaction System at 200 C for 15 min. Digested samples were cooled to 40°C and diluted to 30 ml with distilled water. Blank was prepared for each cycle of digestion using distilled water, nitric acid, and hydrogen peroxide mixture. All the chemicals were trace-element free.
Before the analysis of metal ions in processed blood and urine samples, calibration standards for each element were prepared from multi-element stock solutions (1000 mg L − 1) in triple distilled water. Detection of Cd, Pb, and Cr was performed using a standard sample introduction setup, whereas for As, the hydride generation sample introduction system was utilized. Online hydride generation for As was achieved with an Enhanced Vapor System sample introduction kit using 0.5% m/v sodium tetrahydroborate (NaBH4) stabilized in 0.5% m/v NaOH and 50% v/v HCl solution. Emission data acquisition was performed using the Qtegra ISDS Software at interference-free wavelengths.
Statistical analysis
Statistical analyses were performed with R version 4.2 (R Foundation for Statistical Computing, Vienna, Austria) and IBM SPSS 26 version. The distribution of data in groups was evaluated with Shapiro-Wilk, kurtosis, skewness, and histograms. Skewed data for three groups was compared with the Kruskal-Wallis test. Subgroup analysis in three groups was performed with pairwise comparisons by Dunn test. Parameters with homogeneous distribution were compared with the chi-square test. Data are presented as %, for categorical variables or as median (Q1-Q3) for continuous variables.
Detection rates for blood and urinary metal levels were calculated. For urine metal levels, all statistical analyses were performed with creatinine-adjusted metal concentrations.
Urine to blood ratio was calculated for all metal levels. Spearman correlation coefficient was used to find the association between blood and urine metal levels of individual metals as well as for the association between different metals both in blood and urine. Correlation of blood and urine As with GFR was also performed.
We performed multinominal regression analysis for significantly different metal level in CKDu cases with respect to CKD and healthy controls. We included age and gender (confounding factors) in the model to see the y independence of association and effect estimate of the factor associated with CKDu. Regression model matrices and goodness-of-fit were also determined by the pseudo R 2 coefficient and Hosmer-Lemeshow goodness-of-fit test.
For all analyses, we have considered a p -value less than 0.05 as statistically significant.
A total of 568 patients who visited Nephrology OPD during the study period were screened for inclusion in the study. Out of these, 66 CKDu and 70 CKD cases were found eligible to enroll in the study. Eight patients withdrew consent in the CKD group, whereas four patients in the CKDu group had uncontrolled blood pressure with a single drug, and two withdrew consent. Finally, 60 CKDu and 62 CKD cases were included in the study for outcome analysis. We have approached 120 relatives of patients attending other OPDs and screened them for eligibility criteria of the healthy control group. Out of these, 60 were eligible, and 54 provided consent for participation in the study.
Demography and lab parameters
The CKD and CKDu subjects were similar in demographics for age and sex. However, healthy subjects were younger (Table 1 ). There was no significant difference between CKDu and CKD with reference to stage V (32 vs. 44, P-0.107).There were 05 diabetic kidney disease 04 CKD due to secondary glomerular disease patients (3-lupus nephritis, 1-FSGS), 12 hypertension-associated renal disease, 01 ADPKD, 36 Chronic glomerulonephritis patients, and 04 Chronic pyelonephritis patients in the CKD group. Use of smoking, Alcohol, and painkillers was similar across the three groups (Table 1 ). A significant difference was found between the three study groups with respect to the source of drinking water (ground or surface water). A significantly higher number of CKDu subjects used surface water as a source of drinking water (Table 1 and Table-S 1 and Fig-S 1 ) and a higher number of CKDu subjects reported pesticide usage. As shown in Table 1 , blood pressures were significantly higher in CKD subjects compared to CKDu and healthy subjects and reflect the inclusion criteria with appropriate patient inclusion in three groups. Both ACR and PCR were also significantly different between CKD and CKDu. The eGFR was calculated based on the CKD-EPI formula and was not significantly different between the CKD and CKDu subjects, however, CKD subjects had lower median eGFR compared to CKDu subjects. The healthy subjects had significantly higher eGFR compared to both groups. HbA1c, were similar across the three groups (Table 1 ).
Analytical results
The urinary and blood levels of As, Cd, Pb, and Cr (Table 2 ) were measured in ppb (micrograms per litre), and median with interquartile ranges were reported. Urinary metal levels were also measured in ppb (micrograms per liter) and then adjusted for urinary dilution by urine creatinine value and were finally expressed as micrograms/grams of urine creatinine (Table 2 ).
Detection limits
The lowest detectable concentrations of various heavy metals analyzed on ICP OES with a signal-to-noise ratio of 1 were as follows: As (193.759 nm) - 0.191 ppb; Cd (214.438 nm) - 0 ppb; Pb (220.353 nm) - 0.822 ppb; Cr (283.563 nm) - 3.156 ppb (Table 2 , Figs-S 2 -S 5 ).
Detection percentage
The number of subjects with blood and urine metal levels above the respective detection limits in each study group is reported in Table 2 .
Urine to blood ratio
A urine/blood ratio for each metal in all study groups was calculated for patients with metal levels above the detection limit. The distribution of urine/blood ratios for all metals is presented in Table 2 . Ratios were different between healthy and subjects with deranged kidney functions i.e. low GFR (CKD and CKDu). Median urine/blood Ratio for As was > 1 in healthy subjects and < 1 in CKD and CKDu, reflecting higher urinary levels compared to blood in healthy and reverse in CKD and CKDu subjects. For Pb, it was < 1 in healthy subjects and > 1 in subjects with CKD and CKDu, reflecting higher blood levels compared to urine in healthy and reverse in CKD and CKDu subjects. For Cd and Cr the ratio were < 1 across all three groups suggesting higher urine levels compared to blood levels.
Correlation
A spearman correlation (ρ) was also performed to see the association between each urine and blood metal and among the metals with each other as well. In CKDu, UAs were negatively associated with BAs (ρ-0.260, p -0.11) and in CKD positively (0.138, p -0.37). There was a positive association between urine and blood levels of As,Pb, and Cr and negative association of urine and blood Cd in CKD. In CKDu, a positive association was found in blood and urine Cd,Pb and Cr. In addition, there was a strong correlation of blood Cd, Pb, and Cr ( p < 0.01) [ρ = 0.68 (BCd and BPb), 0.88 (BCd and BCr), 0.71 (BPb and BCr) in CKDu and [ρ = 0.55 (BCd and BPb), 0.82 (BCd and BCr), 0.65 (BPb and BCr) in CKD. The Urine Cd, Pb, and Cr also had strong correlations [ρ = 0.33 (UCd and UPb), and 0.48(UPb and UCr)] in CKD and [ρ = 0.19(UCd and UPb), 0.67 (UCd and UCr), and 0.69 (UPb and UCr)] in CKDu < 0.05 (Table-S 2 -S 4 and Fig-S 6 ). Association of Blood and urine As with GFR was also evaluated, and BAs were found to be negatively associated with GFR (ρ = -0.097, p = 0.56), whereas UAs were positively associated (ρ = 0.14, p = 0.25) with GFR (Table-S 5 ). Metal levels: Blood As: was significantly higher in CKDu ( n = 37) subjects compared to CKD ( n = 41) and healthy ( n = 53) subjects (Table 2 ). On the other hand, the urinary As (UAs) was significantly low in CKD ( n = 50) and CKDu ( n = 48) subjects compared to healthy subjects ( n = 38) and was non significantly higher in CKD subjects compared to CKDu subjects (Fig. 1 , Table 2 ).The blood and urine As values were below detection limits in 21.6%, 35.7%, and 18.8% and in 6%, 16.6%, and 0% of subjects in CKDu, CKD, and healthy groups, respectively.
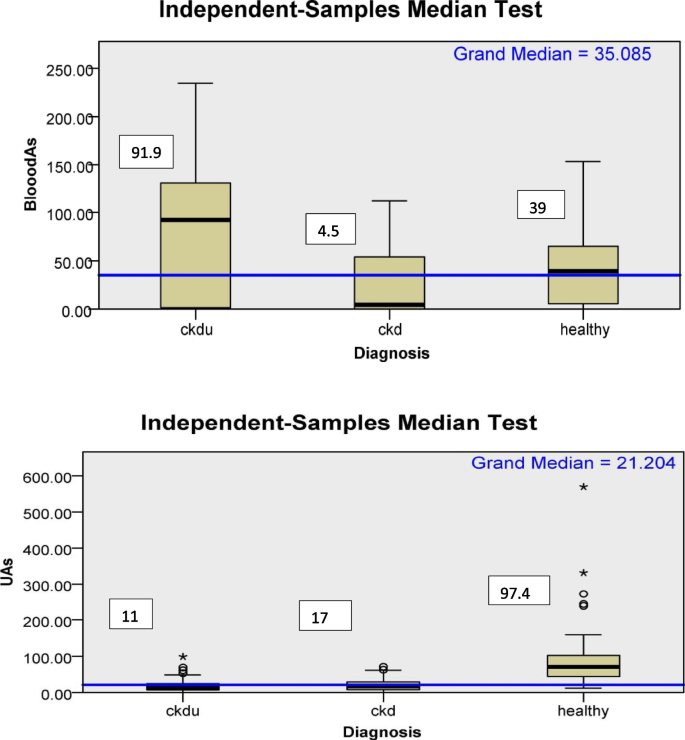
Box plot for distribution of blood and urine arsenic according to diagnosis categories. Median; microgram/Lt (blood); microgram/gm(urine); UAs- Urine arsenic;CKDu-Chronic kidney disease of unknown cause; CKD-Chronic kidney disease
Blood Cd also was significantly higher in CKD and CKDu subjects compared to healthy subjects. Urinary Cd (UCd) levels were significantly higher in CKD and CKDu subjects compared to healthy subjects,. There was a weak association of ( p = 0.06) UCd with CKD subjects compared to CKDu subjects.UCd was higher in CKD subjects compared to CKDu (Fig. 2 , Table 2 ). The blood and urine Cd values were below detection limits in 8.3%, 8.1%, and 0% and 37.2%, 19.3%, and 75.9% of subjects in CKDu, CKD, and healthy groups, respectively.
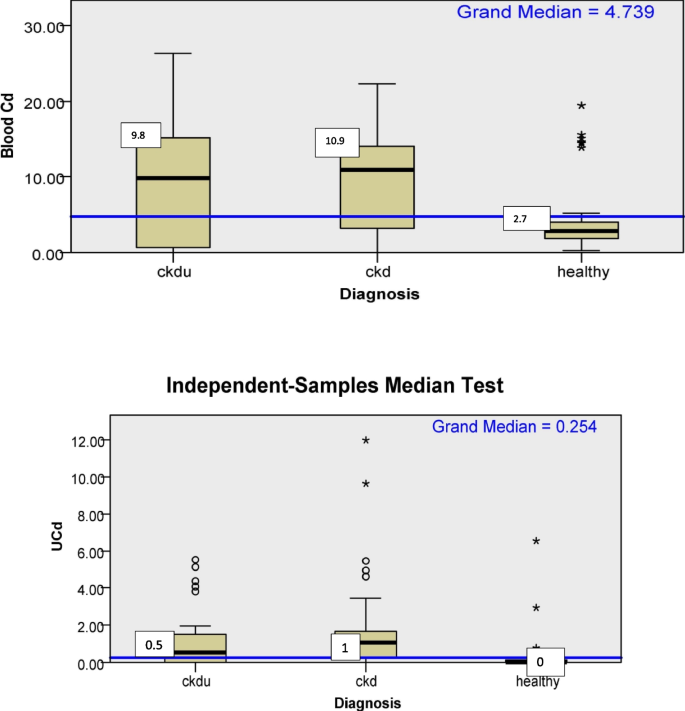
Box plot for distribution of blood and urine cadmium according to diagnosis categories. Median, microgram/Lt (blood); micrograms/gm (urine); UCd- Urine cadmium;CKDu-Chronic kidney disease of unknown cause; CKD-Chronic kidney disease
Pb levels in the blood of CKD and CKDu as well as in urine of CKD and CKDu subjects were significantly higher compared to healthy subjects. The Pb levels were higher in CKD subjects compared to CKDu subjects, but it was not statistically significant (Fig. 3 , Table 2 ). The blood and urine Pb values were below detection limits in 15%, 20%, and 25.9% and 23.3%, 19.4% and 70.4% of subjects in CKDu, CKD, and healthy groups, respectively.
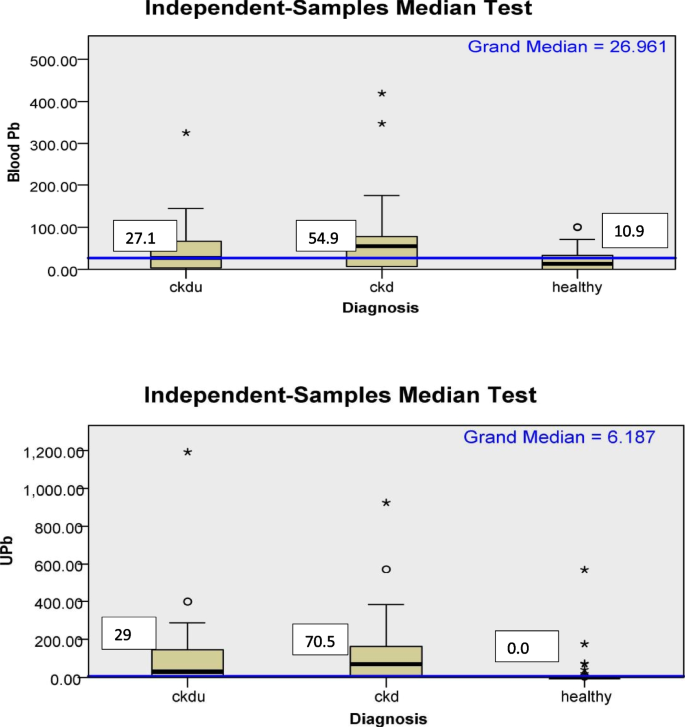
Box plot for distribution of blood and urine lead according to diagnosis categories. microgram/Lt (blood); microgram/gm(urine); UPb- Urine lead;CKDu-Chronic kidney disease of unknown cause; CKD-Chronic kidney disease
As shown in Table 2 and Fig. 4 , urinary and blood Cr was significantly higher in CKD, and CKDu patients than healthy subjects. The blood and urine Cr values were below detection limits in 13%, 0%, and 0% and 13.3%, 14.5% and 85.2% of subjects in CKDu, CKD, and healthy groups, respectively.
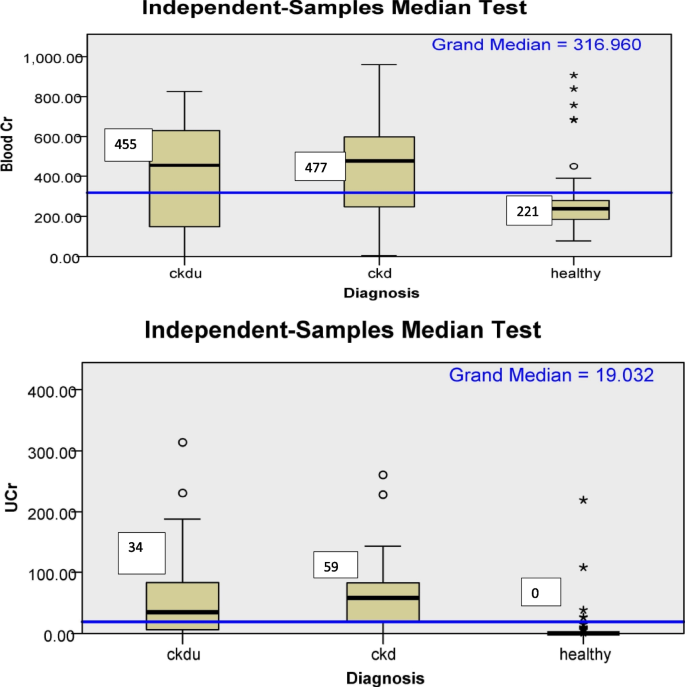
Box plot for distribution of blood and urine chromium according to diagnosis categories. Median; microgram/Lt(blood); microgram/gm(urine); UCr-urine chromium, CKDu-Chronic kidney disease of unknown cause; CKD-Chronic kidney disease
Multinominal regression
Though age, gender, were not significantly different between CKDu and CKD, on univariate analysis, we included these In the multinominal regression analysis between CKDu and CKD in reference to healthy subjects in addition to factors found significantly different ( p < 0.01 on univariate analysis) i.e. blood As and source of drinking water.After the final model, gender had no association with CKDu. Blood As, surface water as drinking water source and age were independently associated with CKDu. Age was associated independently with CKD also (Table 3 ).
To the best of our knowledge, this is the first study wherein an attempt has been made to analyze the association of heavy metals with CKDu in central India, using blood and urine levels as biomarkers of metal exposure. In addition, CKD and healthy subjects have been used as control groups.
The current study showed that blood and urine creatinine-adjusted urinary levels of heavy metals Cd, Pb and Cr were significantly higher in patients with CKD and CKDu as compared to healthy subjects. The urinary levels of the above metals were undetectable in healthy subjects. The study also showed a weak association of ( p = < 0.06) higher urinary Cd in CKD subjects compared to CKDu subjects of this Indian cohort.
The study also showed that Blood As was significantly higher in CKDu subjects compared to CKD and healthy subjects. On multinominalregression, blood As was independently ( p < 0.05) associated with CKDu after age adjustment.
In our study, median GFR was rather high in CKDu subjects [14.5 (7.0, 34.2)] compared to GFR in CKD subjects [9.0 (6.0, 17.0)ml/min/1.73m 2 )] and it was non significantly different between the two groups. On correlation analysis, there was a negative correlation between Blood As and GFR and a positive correlation of urine As with GFR. Based on this, the higher blood As in CKDu with higher GFR appears to be truly elevated.
Previously a study from Sri Lanka has also reported an association of CKDu with chronic As toxicity. In that study, 48% of CKDu patients and 17.4% of the control subjects fulfilled the criteria to be diagnosed with chronic arsenical toxicity(CAT), indicating the potential link between CAT and CKDu and suggesting agrochemicals could be the possible source [ 14 ]. Later, it was reported that glyphosate was the most widely used pesticide in Sri Lanka, which contains an average of 1.9 mg/kg arsenic. Findings suggest that agrochemicals, especially phosphate fertilizers, are a major source of inorganic arsenic in CKDu endemic areas [ 15 ]. However, another study from Sri Lanka did not find any difference in UAs levels in patients of CKDu in endemic areas and controls from endemic and nonendemic areas [ 4 ].
Some other studies have reported associations of As with CKD. A study from Taiwan found total UAs to be associated with a four-fold risk of CKD [ 6 ]. Another study reported an association of MMA V (mono methyl arsenate pentavalent) and DMA V (dimethyl arsenate pentavalent) in urine with prevalence of CKD [ 16 ]. However, in both studies, the type of CKD was not reported.
The higher blood As in CKDu compared to CKD may be associated with exposures in our study; a significantly higher number of subjects in CKDu group reported use of pesticides, surface water as a source of drinking water in CKDu subjects.On regression analysis also, surface water was independently associated with CKDu.
A study from north India reported increased levels of OCPs, namely α-HCH, aldrin, and β-endosulfan, in CKDu patients as compared to healthy control and CKD patients of known etiology [ 17 ] and it is also known that arsenic is an important component of pesticides [ 18 ]. The contamination of surface water with various pollutants i.e. pesticides, is common [ 19 ]. Arsenic is a known nephrotoxin, and one of the case reports where kidney histopathology was evaluated reported As causes tubulointerstitial disease (TID) [ 20 ]. The difference in methylation processes of As has also been found responsible for various diseases associated with As i.e. for example, high proportions of urinary MMAs (%U-MMAs) have been associated with a higher risk of cancers and skin lesions [ 21 ]. In contrast, high %U-DMAs has been associated with diabetes risk [ 22 ]. We have measured only iAs in our study. Whether methylation resulting in various metabolite species has different associations with CKDu or CKD should be explored further. We recently found a significant association of single nucleotide polymorphism in a gene coding for sodium-dependent dicarboxylate transporter (SLC13A3) with the susceptibility to CKDu [ 23 ].
In the current study, the UAs results suggest that As levels of 97 µg/gm of creatinine in healthy subjects were not associated with decreased GFR or proteinuria. Similar results were reported by a study from China where researchers found a lower confidence limit on the benchmark dose (LBMD) of 102 and 0.88 µg/gm creatinine for As and Cd, respectively, in order to prevent renal damage in the general population co-exposed to arsenic and cadmium [ 24 ]. The UAs in healthy subjects in our study were nearly similar to the LBMD reference and, not surprisingly, not to be associated with CKD or proteinuria.
Some studies have reported lead to be associated with CKDu. An Indian study reported high levels of lead and silicon concentrations in Indian groundwater in the endemic Uddanam area [ 7 ]. Jaysuman et al. also reported higher levels of Pb (26.5 µg/gm) in the urine of patients with Sri Lankan agricultural nephropathy compared to endemic and nonendemic control [ 25 ].
In the current study, although the median level of blood Pb was almost double in CKD patients compared to CKDu, the result was not statistically significant.
Our study showed that Cd was significantly associated with renal disease. Blood Cd and urine Cd (UCd) levels were significantly higher in patients with renal disease (CKD and CKDu) as compared to healthy subjects. The findings of UCd also showed a weak association (p-0.06) of Cd with CKD compared to CKDu among patients with renal diseases. There are some concerns that UCd may not be truly reflective of metal burden in patients with advanced CKD [ 26 ], because initially, in the course of Cd toxicity with early tubular damage, the normal reabsorption of cadmium-metallothionein decreases, and the UCd concentration increases. However, in the long run, cadmium-induced kidney damage gives rise to low Cd concentrations in both the kidney and urine, while the tubular damage remains [ 27 ]. The U/B ratio of < 1 for Cd in our study supports the above findings.
The mean eGFR in our CKD cohort was lower compared to CKDu; despite this, higher UCd values in patients with CKD compared to CKDu in our study indicate a potential association of Cd with CKD.
Studies have reported variable association of Cd with CKDu when compared to healthy subjects. Nanayakkara et al. [ 28 ] did not find an association of UCd with CKDu in stages 1–4 compared to healthy controls. Whereas another Sri Lankan [ 4 ] study found significantly high UCd in patients with CKDu against the endemic and nonendemic controls. We also observed significantly higher UCd in CKDu vs. healthy controls.
In the current study, urinary Cr (UCr) was not detected in healthy subjects, whereas it was significantly higher in patients with CKD and CKDu as compared to healthy subjects. UCr levels were higher in CKD compared to CKDu. Epidemiologically, Cr exposure has been reported to be associated with kidney damage in occupational populations [ 26 ]. Recently, a study from Taiwan reported that a significant and independent association between Cr exposure and decreased renal function in the general population, and co-exposure to Cr with Pb and Cd is potentially associated with an additional decline in the GFR in Taiwanese adults [ 27 ]. A study from Bangladesh reported outcomes similar to our study; however, the study included only CKD ( n = 30) patients and compared them with healthy subjects ( n = 20). In that study, compared to the controls, CKD patients exhibited significantly higher levels of Pb, Cd, and Cr levels in their urine samples. This signifies a potential association between heavy metal co-exposure and CKD [ 29 ]. In the current study a significant correlation between blood Cd, Pb, and Cr and urine Cd, Pb, and Cr were found in CKDu and CKD subjects compared to healthy subjects. The levels of UCd, UPb, and UCr in CKD and CKDu patients were significantly higher compared to healthy controls; The possibility of the combined effect of Cd, Pb, and Cr in the causation of renal diseases could be evaluated further in future studies. As CKDu is an endemic disease, the results of our study suggest an association of arsenic with CKDu in the Indian population, and so the generalizability of the result should be used with caution.
Strengths and limitations
This is the first study which has included two controls (CKD and healthy) and compared metal levels in patients with CKDu. In addition, the comparison of metals in both blood and urine is another advantage, as falling GFR levels and urine levels of several metals do not reflect true metal burden in patients. Inclusion of CKDu patients, as per the suggested definition by the Indian society of Nephrology, is another strength of our study.
The small sample size of our study may be a limitation of our study though it was calculated scientifically. The study involved Indian patients and controls only so the generalization of the results should be with caution. Healthy controls were of younger age is also a limitation of the study.
Also the study included patients from central India, comparatively a larger area and does not points out endemicity.
The study finds an association of environmental toxins with CKDu and CKD. The age and sex-adjusted As were observed to have an independent association with CKDu. A weak association of Cd with CKD was also observed in this Indian cohort. Subjects with renal dysfunction (CKDu and CKD) were observed to have a significantly higher metal burden of Pb, Cd, As, and Cr as compared to healthy controls. CKDu patients may have higher exposure to As via pesticides, surface water usage, or both.
Availability of data and materials
The datasets used and/or analysed during the current study are available from the corresponding author on reasonable request.
Abbreviations
Chronic Kidney Disease of unknown cause
Chronic Kidney Disease
Kidney Disease Improving Global Outcomes
Glomerular filteration rate
Institutional Human Ethics committee
Concentrated trace metal grade nitric acid
Hydrogen peroxide
High-purity polytetrafluoroethylene
Inorganic As
Tubulo-interstitial disease
Pentavalent monomethylarsonic acid
Pentavalent dimethylarsinic acid
Methylarsenous acid
Sodium-dependent dicarboxylate transporter
Limit on the benchmark dose
Arsenobetaine
Urinary MMAs
Urinary DMAs
Blood lead levels
End-stage kidney disease
Chapter 1: definition and classification of CKD. Kidney Int Suppl (2011). 2013;3(1):19–62. https://doi.org/10.1038/kisup.2012.64 .
Gifford FJ, Gifford RM, Eddleston M, Dhaun N. Endemic nephropathy around the world. Kidney Int Rep. 2017;2(2):282–92. https://doi.org/10.1016/j.ekir.2016.11.003 .
Article PubMed Google Scholar
Nanayakkara S, Senevirathna S, Parahitiyawa NB, et al. Whole-exome sequencing reveals genetic variants associated with chronic kidney disease characterized by tubulointerstitial damages in North Central Region, Sri Lanka. Environ Health Prev Med. 2015;20(5):354–9. https://doi.org/10.1007/s12199-015-0475-1 .
Article CAS PubMed PubMed Central Google Scholar
Jayatilake N, Mendis S, Maheepala P, Mehta FR. Chronic kidney disease of uncertain aetiology: prevalence and causative factors in a developing country. BMC Nephrol. 2013;14(1):180. https://doi.org/10.1186/1471-2369-14-180 .
Article PubMed PubMed Central Google Scholar
Jayasumana C, Gunatilake S, Senanayake P. Glyphosate, hard water and nephrotoxic metals: are they the culprits behind the epidemic of chronic kidney disease of unknown etiology in Sri Lanka? Int J Environ Res Public Health. 2014;11(2):2125–47. https://doi.org/10.3390/ijerph110202125 .
Bawaskar HS, HimmatraoBawaskar P, Himmatrao BP. Chronic renal failure associated with heavy metal contamination of drinking water: a clinical report from a small village in Maharashtra. Clin Toxicol. 2010;48(7):768–768. https://doi.org/10.3109/15563650.2010.497763 .
Article Google Scholar
Lal K, Sehgal M, Gupta V, et al. Assessment of groundwater quality of CKDu affected Uddanam region in Srikakulam district and across Andhra Pradesh, India. Groundw Sustain Dev. 2020;11:100432. https://doi.org/10.1016/j.gsd.2020.100432 .
Zhang X, Cornelis R, de Kimpe J, et al. Accumulation of arsenic species in serum of patients with chronic renal disease. Clin Chem. 1996;42(8):1231–7. https://doi.org/10.1093/clinchem/42.8.1231 .
Article CAS PubMed Google Scholar
Bommarito PA, Beck R, Douillet C, et al. Evaluation of plasma arsenicals as potential biomarkers of exposure to inorganic arsenic. J Expo Sci Environ Epidemiol. 2019;29(5):718–29. https://doi.org/10.1038/s41370-019-0121-x .
Environmental Health Criteria 165. Joint sponsorship of The United Nations Environment Programme, The International Labour Organization, and The World Health Organization. 1995. https://apps.who.int/iris/bitstream/handle/10665/37241/9241571659-eng.pdf?sequence=1 . Accessed 10 Nov 2022.
Ashrap P, Watkins DJ, Mukherjee B, et al. Predictors of urinary and blood Metal(loid) concentrations among pregnant women in Northern Puerto Rico. Environ Res. 2020;183:109178. https://doi.org/10.1016/j.envres.2020.109178 .
Anupama Y, Sankarasubbaiyan S, Taduri G. Chronic kidney disease of unknown etiology: case definition for India – a perspective. Indian J Nephrol. 2019. https://doi.org/10.4103/ijn.IJN_327_18 .
Levey AS, Stevens LA, Schmid CH, et al. CKD-EPI (Chronic Kidney Disease Epidemiology Collaboration). A new equation to estimate glomerular filtration rate. Ann Intern Med. 2009;150(9):604–12. https://doi.org/10.7326/0003-4819-150-9-200905050-00006 .
Jayasumana MACS, Paranagama PA, Amarasinghe KMRC, et al. Possible link of chronic arsenic toxicity with chronic kidney disease of unknown etiology in Sri Lanka. J Nat Sci Res. 2013;3(1). www.iiste.org ISSN 2224-3186 (Paper) ISSN 2225-0921 (Online).
Jayasumana C, Fonseka S, Fernando A, et al. Phosphate fertilizer is a main source of arsenic in areas affected with chronic kidney disease of unknown etiology in Sri Lanka. Springerplus. 2015;4:90. https://doi.org/10.1186/s40064-015-0868-z .
Zheng LY, Umans JG, Yeh F, et al. The association of urine arsenic with prevalent and incident chronic kidney disease. Epidemiology. 2015;26(4):601–12. https://doi.org/10.1097/EDE.0000000000000313 .
Ghosh R, Siddarth M, Singh N, et al. Organochlorine pesticide level in patients with chronic kidney disease of unknown etiology and its association with renal function. Environ Health Prev Med. 2017;22(1):49. https://doi.org/10.1186/s12199-017-0660-5 . Published 2017 May 26.
Li Y, Ye F, Wang A, et al. Chronic arsenic poisoning probably caused by arsenic-based pesticides: findings from an investigation study of a household. Int J Environ Res Public Health. 2016;13(1):133. https://doi.org/10.3390/ijerph13010133 .
Agrawal A, Pandey R, Sharma B. Water pollution with special reference to pesticide contamination in India. J Water Resource Prot. 2010;2(5):432–48. https://doi.org/10.4236/jwarp.2010.25050 .
Prasad GVR, Rossi NF. Arsenic intoxication associated with tubulointerstitial nephritis. Am J Kidney Dis. 1995;26(2):373–6. https://doi.org/10.1016/0272-6386(95)90660-6 .
Kuo CC, Moon KA, Wang SL, Silbergeld E, Navas-Acien A. The association of arsenic metabolism with cancer, cardiovascular disease, and diabetes: a systematic review of the epidemiological evidence. Environ Health Perspect. 2017;125(8):087001. https://doi.org/10.1289/EHP577 .
del Razo LM, García-Vargas GG, Valenzuela OL, et al. Exposure to arsenic in drinking water is associated with increased prevalence of diabetes: a cross-sectional study in the Zimapán and Lagunera regions in Mexico. Environ Health. 2011;10(1):73. https://doi.org/10.1186/1476-069X-10-73 .
Kumari R, Tiwari S, Atlani M, et al. Association of single nucleotide polymorphisms in KCNA10 and SLC13A3 genes with the susceptibility to chronic kidney disease of unknown etiology in central Indian patients [published online ahead of print, 2023 Jan 25]. Biochem Genet. 2023. https://doi.org/10.1007/s10528-023-10335-7 .
Hong F, Jin T, Zhang A. Risk assessment on renal dysfunction caused by co-exposure to arsenic and cadmium using benchmark dose calculation in a Chinese population. Biometals. 2004;17(5):573–80. https://doi.org/10.1023/B:BIOM.0000045741.22924.d .
Jayasumana C, Gunatilake S, Siribaddana S. Simultaneous exposure to multiple heavy metals and glyphosate may contribute to Sri Lankan agricultural nephropathy. BMC Nephrol. 2015;16(1):103. https://doi.org/10.1186/s12882-015-0109-2 .
Wilbur S, Abadin H, Fay M, et al. Toxicological profile for chromium. Atlanta: Agency for Toxic Substances and Disease Registry (US); 2012.
Google Scholar
Tsai TL, Kuo CC, Pan WH, et al. The decline in kidney function with chromium exposure is exacerbated with co-exposure to lead and cadmium. Kidney Int. 2017;92(3):710–20. https://doi.org/10.1016/j.kint.2017.03.013 .
Nanayakkara S, Senevirathna STMLD, Karunaratne U, et al. evidence of tubular damage in the very early stage of chronic kidney disease of uncertain etiology in the North Central Province of Sri Lanka: a cross-sectional study. Environ Health Prev Med. 2012;17(2):109–17. https://doi.org/10.1007/s12199-011-0224-z .
Choudhury TR, Zaman SKZ, Chowdhury TI, Begum BA, Islam MDA, Rahman MDM. Status of metals in serum and urine samples of chronic kidney disease patients in a rural area of Bangladesh: an observational study. Heliyon. 2021;7(11):e08382. https://doi.org/10.1016/j.heliyon.2021.e08382 .
Download references
Acknowledgements
Not applicable.
The Study was funded by Indian council of Medical Research (ICMR),New Delhi, India. Sanction no.:5/4/7-14/2019-NCD-II.
Author information
Mahendra Atlani and Ashok Kumar contributed equally to this work.
Authors and Affiliations
Department of Nephrology, All India Institute of Medical Sciences (AIIMS), Room No-3022, Academic Block, 3rd Floor, Saket Nagar, Bhopal, Madhya Pradesh, 462020, India
Mahendra Atlani, M. N. Meenu & Athira Anirudhan
Department of Biochemistry, All India Institute of Medical Sciences (AIIMS), Saket Nagar, Bhopal, Madhya Pradesh, 462020, India
Ashok Kumar, Sudhir K. Goel & Ravita Kumari
Department of Environmental Biochemistry, ICMR-National Institute for Research in Environmental Health (NIREH), Bhopal, Madhya Pradesh, India
Rajesh Ahirwar
All India Institute of Medical Sciences (AIIMS), Bhopal, Madhya Pradesh, India
Saikrishna Vallamshetla & G. Sai Tharun Reddy
You can also search for this author in PubMed Google Scholar
Contributions
MA and AK were equally involved in conceptualising the study, winning the grant, analyzing the results, monitoring the progress of study. MA prepared the manuscript. AK and SKG did the editing. RA supervised analysis of metal levels, sample collection done by MMN, RK. Metal analysis done by AA. Data entry and file preparation for results done by MMN, AA, SKV and STR.
Corresponding authors
Correspondence to Mahendra Atlani or Ashok Kumar .
Ethics declarations
Ethics approval and consent to participate.
The study was approved by the Institutional Human Ethics Committee (IHEC) approval No- EF0125. The study was performed according to the guidelines of the Declaration of Helsinki. All participants gave informed consent for participation before enrolment.
Consent for publication
Competing interests.
The authors declare no competing interests.
Additional information
Publisher’s note.
Springer Nature remains neutral with regard to jurisdictional claims in published maps and institutional affiliations.
Supplementary Information
Supplementary material 1., rights and permissions.
Open Access This article is licensed under a Creative Commons Attribution 4.0 International License, which permits use, sharing, adaptation, distribution and reproduction in any medium or format, as long as you give appropriate credit to the original author(s) and the source, provide a link to the Creative Commons licence, and indicate if changes were made. The images or other third party material in this article are included in the article's Creative Commons licence, unless indicated otherwise in a credit line to the material. If material is not included in the article's Creative Commons licence and your intended use is not permitted by statutory regulation or exceeds the permitted use, you will need to obtain permission directly from the copyright holder. To view a copy of this licence, visit http://creativecommons.org/licenses/by/4.0/ . The Creative Commons Public Domain Dedication waiver ( http://creativecommons.org/publicdomain/zero/1.0/ ) applies to the data made available in this article, unless otherwise stated in a credit line to the data.
Reprints and permissions
About this article
Cite this article.
Atlani, M., Kumar, A., Ahirwar, R. et al. Heavy metal association with chronic kidney disease of unknown cause in central India-results from a case-control study. BMC Nephrol 25 , 120 (2024). https://doi.org/10.1186/s12882-024-03564-4
Download citation
Received : 28 April 2023
Accepted : 26 March 2024
Published : 03 April 2024
DOI : https://doi.org/10.1186/s12882-024-03564-4
Share this article
Anyone you share the following link with will be able to read this content:
Sorry, a shareable link is not currently available for this article.
Provided by the Springer Nature SharedIt content-sharing initiative
- Chronic kidney disease of unknown cause
- Heavy metals
- Environmental toxins
BMC Nephrology
ISSN: 1471-2369
- Submission enquiries: [email protected]
- General enquiries: [email protected]
- Milano Cortina 2026
- Brisbane 2032
- Olympic Refuge Foundation
- Olympic Games
- Olympic Channel
- Let's Move
Race for IPL 2024 Purple Cap: The bowlers with the most wickets
CSK’s Mustafizur Rahman currently leads the race for the IPL 2024 Purple Cap. Know list of top wicket takers.
The race for the top wicket-taker in the Indian Premier League ( IPL ) 2024 is heating up.
The league's top wicket-taker at the end of the season is presented the Purple Cap award and some of the best foreign players and promising Indian bowlers are competing for the honour in IPL 2024 .
With seven wickets from three games, Chennai Super Kings’ Mustafizur Rahman currently holds the Purple Cap in IPL 2024 .
The players with the most wickets during the tournament wear the Purple Cap while on the field. However, the bowler with the most wickets at the end of the tournament will win the Purple Cap award. In case of a tie, the bowler with the better economy rate ranks higher on the list.
A total of 14 bowlers have had the honour of winning the Purple Cap at the end of the season from the previous 16 editions. Dwayne Bravo, playing for CSK in 2013 and 2015, and Bhuvneshwar Kumar, for Sunrisers Hyderabad in 2016 and 2017, are the two bowlers who have won the Purple Cap twice.
Bravo’s 32 wickets in 2013 is the joint-most number of wickets any bowler has taken in any single edition. Harshal Patel, playing for RCB, matched the tally in 2021.
Rajasthan Royals’ Sohail Tanvir took 22 wickets in 11 matches at the inaugural edition in 2008 and was the first player to win the Purple Cap in the IPL.
Interestingly, only three times the highest wicket-takers have come from the teams who eventually became champions - Rajasthan Royals’ Sohail Tanvir in 2008, Deccan Chargers’ RP Singh in 2009 and Sunrisers Hyderabad’s Bhuvneshwar Kumar in 2016.
The IPL 2024 began on March 22 and the final will be held at the MA Chidambaram Stadium in Chennai on May 26.
Here’s an updated list of which player has taken the most wickets in IPL 2024.
Race for Purple Cap IPL 2024: Most wickets in a season
Related content
Impact Player rule in IPL explained
What is Net Run Rate in cricket and how to calculate it - formulae and case studies
You may like.
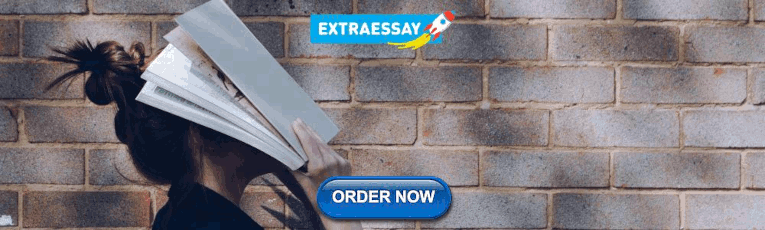
IMAGES
VIDEO
COMMENTS
Air quality impacts of landfill fires: A case study from the Brahmapuram Municipal Solid Waste Treatment Plant in Kochi, India. ... 2020), and for the Deonar landfill of Mumbai, India (Average FRP = 23.8 MW), it was several times higher than that of BMSWTP for a period from 2011 to 2017 (Sharma et al., 2019).
Smahi D, Hammoumi OE, Fekri A (2013) Assessment of the impact of the landfill on groundwater quality: a case study of Mediouna site, Casablanca, Morocco. J Water Resour Prot 5:440-445. ... (2014) Characterization and management of municipal solid waste: a case study of Varanasi city, India. Int J Curr Res Acad Rev 2:10-16.
Challenges, and Recommendations. Swati 1 , Indu Shekhar Thakur1 , Virendra Kumar Vijay2 and Pooja Ghosh2. (1)School of Environmental Sciences, Jawaharlal Nehr u University, New Delhi, India. (2 ...
The authors of all reviewed papers propose a landfill siting methodology and conduct their research on a case study. Table 1 presents the country where the case study was carried out, and the frequency/total number of publications per country out of total number of identified 30 case study countries. Iran, Turkey, India, Greece and USA and are the top five countries rated according to the ...
Kolkata is one of the four major metropolitan cities in India and the capital city of the state of West Bengal. With an area of 187.33 km 2 and a population of about 10 million (including a floating population of about 6 million), the city generates about 3500 Metric Ton (MT) of solid waste per day. Currently, Kolkata Municipal Corporation (KMC ...
The application of the new risk assessment method is demonstrated with a case study for a landfill located within a heavily populated area of New Delhi, India. Introduction Stability of mega-landfills became headline news in 1977 when a large landfill in Sarajevo, Yugoslavia, failed, causing the translational movement of 200,000 m 3 of ...
A case study on establishing the state of decomposition of municipal solid waste in a bioreactor landfill in India. Arif Mohammad, Piotr ... Yamin L, et al. (2002) The landslide of Dona Juana landfill in Bogota. A case study. In: Proceedings of the fourth international congress on environmental geotechnics (4th ICEG), Rio de Janeiro, Brazil, 11 ...
The first case study highlights enhancement of capacity by design, construction and stability analysis of a new (third) phase of HW landfill, which makes use of the existing air space between two completed (mound-shaped) phases of the HW landfill. The second case study pertains to increasing the height of a landfill from 14 to 31.8 m, and ...
This study aims to understand groundwater contamination due to leachate leaks at several municipal solid waste landfill sites in India and their possible impacts on human health and environment ...
ISSN 2348 - 8034 Impact Factor- 4.022 [Chaitanaya *, 4(10): October 2017] GLOBAL JOURNAL OF ENGINEERING SCIENCE AND RESEARCHES IMPACT ON ENVIRONMENT BY LANDFILL AND A CASE STUDY ON VISHAKHAPATNAM LANDFILL D. V. SK Chaitanaya*1 & P. Neeharika2 Dept. of Civil Engineering, Acharya Nagarjuna University Guntur , India *1&2 ABSTRACT Solid waste ...
Selection of Solid Waste Landfills for Developing Townships in India: A GIS Based Case Study. Download Free PDF View PDF. Solid Waste Disposal Site Selection for Bareilly District, India Integrating GIS And Risk Assessment ... Kara, C., and N. Doratli. 2012. Application of GIS/AHP in siting sanitary landfill: A case study in Northern Cyprus ...
Case studies related t o biomining of landfi lls, India: In . India landfill mining was observed in cities with closed or . overflowing landfills. In 2002-03, well-documented .
Urban India accounts for a third of India's population and generates 54.75 million tonne of MSW annually. From 2014 onwards, the Swachh Bharat Mission has been emphasising on reclamation of landfill sites to adhere to the Solid Waste Management (SWM) Rules, 2016, and the guidelines of the Hon National Green Tribunal, with an aim to recover ...
A. Ohri, P.K. Singh, Landfill site selection using site sensitivity index a case study of Varanasi city in India, in, Proceedings of 1st International Conference on Solid Waste Management. KhudiramAnushilan Kendra (KAK) and Netaji Indoor Stadium (NIS) , Kolkata, India, pp. 31-38 (2009)
In another study performed on the emission of GHG from a landfill site by Sing and et al. (2016) in Delhi India, the results showed that urban waste disposal is the most important source of GHG emission. ... Pazoki M. Estimation and modeling of gas emissions in municipal landfill (Case study: Landfill of Jiroft City) Pollution. 2017; 3 (4):689 ...
New Delhi CNN —. At the Bhalswa landfill in northwest Delhi, a steady flow of jeeps zigzag up the trash heap to dump more garbage on a pile now over 62 meters (203 feet) high. Fires caused by ...
The cities of Bobbili (Andhra Pradesh), Mysuru (Karnataka) and Vengurla (Maharashtra) found mention for handling biodegradable waste. Zero waste plants in Mysuru, in particular, scripted its success. Leh (Ladakh) installed a 30 tonne solar waste segregation plant, earning its spot among the cities that use technology to solve waste management.
Beside that green house gases (GHG) emission from landfill areas is concern to the global warming. In the present study the methane and nitrous oxide were analysed in Ghazipur landfill area of ...
Kerala's top court said it will take up the case on Tuesday. ... the study added, of which only about 1% is suitable for recycling. ... the landfill is not India's largest. The Deonar dumping ...
This paper discusses the effects of a potential leachate leakage from a municipal solid waste landfill, situated at Mavallipura, Bangalore, India, on the surrounding water bodies. The landfill area is spread over an area of about 100 acres that began accepting waste from 2005. MSW was deposited in non-engineered manner that has resulting in steep and unstable slopes, leachate accumulation ...
Firefighters battle the blaze at the Ghazipur landfill on March 28, 2022 in New Delhi, India. Firefighters in India's capital said Wednesday they are close to extinguishing a fire at a landfill ...
Background Chronic Kidney Disease of unknown cause (CKDu) a disease of exclusion, and remains unexplained in various parts of the world, including India. Previous studies have reported mixed findings about the role of heavy metals or agrochemicals in CKDu. These studies compared CKDu with healthy controls but lacked subjects with CKD as controls. The purpose of this study was to test the ...
The race for the top wicket-taker in the Indian Premier League (IPL) 2024 is heating up.. The league's top wicket-taker at the end of the season is presented the Purple Cap award and some of the best foreign players and promising Indian bowlers are competing for the honour in IPL 2024.. With seven wickets from three games, Chennai Super Kings' Mustafizur Rahman currently holds the Purple Cap ...