
An official website of the United States government
The .gov means it’s official. Federal government websites often end in .gov or .mil. Before sharing sensitive information, make sure you’re on a federal government site.
The site is secure. The https:// ensures that you are connecting to the official website and that any information you provide is encrypted and transmitted securely.
- Publications
- Account settings
Preview improvements coming to the PMC website in October 2024. Learn More or Try it out now .
- Advanced Search
- Journal List
- Neuropsychiatr Dis Treat
- v.3(5); 2007 Oct
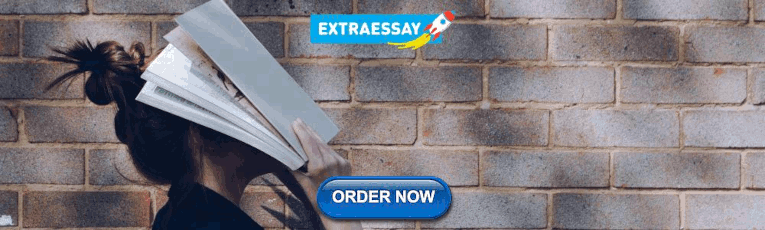
Sleep deprivation: Impact on cognitive performance
Paula alhola.
1 Department of Psychology
Päivi Polo-Kantola
2 Sleep Research Unit (Department of Physiology), University of Turku, Turku, Finland
Today, prolonged wakefulness is a widespread phenomenon. Nevertheless, in the field of sleep and wakefulness, several unanswered questions remain. Prolonged wakefulness can be due to acute total sleep deprivation (SD) or to chronic partial sleep restriction. Although the latter is more common in everyday life, the effects of total SD have been examined more thoroughly. Both total and partial SD induce adverse changes in cognitive performance. First and foremost, total SD impairs attention and working memory, but it also affects other functions, such as long-term memory and decision-making. Partial SD is found to influence attention, especially vigilance. Studies on its effects on more demanding cognitive functions are lacking. Coping with SD depends on several factors, especially aging and gender. Also interindividual differences in responses are substantial. In addition to coping with SD, recovering from it also deserves attention. Cognitive recovery processes, although insufficiently studied, seem to be more demanding in partial sleep restriction than in total SD.
Introduction
A person’s quality of life can be disrupted due to many different reasons. One important yet underestimated cause for that is sleep loss ( National Sleep Foundation 2007 ). Working hours are constantly increasing along with an emphasis on active leisure. In certain jobs, people face sleep restriction. Some professions such as health care, security and transportation require working at night. In such fields, the effect of acute total sleep deprivation (SD) on performance is crucial. Furthermore, people tend to stretch their capacity and compromise their nightly sleep, thus becoming chronically sleep deprived.
When considering the effects of sleep loss, the distinction between total and partial SD is important. Although both conditions induce several negative effects including impairments in cognitive performance, the underlying mechanisms seem to be somewhat different. Particularly, results on the recovery from SD have suggested different physiological processes. In this review, we separately consider the effects of acute total and chronic partial SD and describe the effects on cognitive performance. The emphasis on acute total SD reflects the quantity of studies carried out compared with partial SD. The effects of aging and gender, as well as interindividual differences are discussed. We concentrate on the studies that have been published since 1990.
Sleep and sleep loss
The need for sleep varies considerably between individuals ( Shneerson 2000 ). The average sleep length is between 7 and 8.5 h per day ( Kripke et al 2002 ; Carskadon and Dement 2005 ; Kronholm et al 2006 ). Sleep is regulated by two processes: a homeostatic process S and circadian process C (eg, Achermann 2004 ). The homeostatic process S depends on sleep and wakefulness; the need for sleep increases as wakefulness continues. The theory for circadian process C suggests a control of an endogenous circadian pacemaker, which affects thresholds for the onset and offset of a sleep episode. The interaction of these two processes determines the sleep/wake cycle and can be used to describe fluctuations in alertness and vigilance. Although revised “three-process models” (eg, Akerstedt and Folkard 1995 ; Van Dongen et al 2003b ; Achermann 2004 ) have been suggested, this classical model is the principal one used for study designs in SD research.
There are many unanswered questions regarding both the functions of sleep and the effects of sleep loss. Sleep is considered to be important to body restitution, like energy conservation, thermoregulation, and tissue recovery ( Maquet 2001 ). In addition, sleep is essential for cognitive performance, especially memory consolidation ( Maquet 2001 ; Stickgold 2005 ). Sleep loss, instead, seems to activate the sympathetic nervous system, which can lead to a rise of blood pressure ( Ogawa et al 2003 ) and an increase in cortisol secretion ( Spiegel et al 1999 ; Lac and Chamoux 2003 ). Immune response may be impaired and metabolic changes such as insulin resistance may occur (for review, see Spiegel et al 2005 ). People who are exposed to sleep loss usually experience a decline in cognitive performance and changes in mood (for meta-analyses, see Pilcher and Huffcutt 1996 ; Philibert 2005 ).
Sleep deprivation is a study design to assess the effects of sleep loss. In acute total SD protocols, the subjects are kept awake continuously, generally for 24–72 hours. In chronic partial SD, subjects are allowed restricted sleep time during several consecutive nights. Although chronic sleep restriction is more common in the normal population and thus offers a more accurate depiction of real life conditions, total SD has been more thoroughly explored.
Cognitive performances measured in SD studies have included several domains. The most thoroughly evaluated performances include different attentional functions, working memory, and long-term memory. Visuomotor and verbal functions as well as decision-making have also been assessed. Sleep deprivation effects on cognitive performance depend on the type of task or the modality it occupies (eg, verbal, visual, or auditory). In addition, task demands and time on task may play a role. The task characteristics are discussed in more detail in following sections where the existing literature on the cognitive effects of SD is reviewed.
Mechanisms behind sleep loss effects
Some hypotheses are proposed to explain why cognitive performance is vulnerable to prolonged wakefulness. The theories can be divided roughly in two main approaches, in which SD is assumed to have (1) general effects on alertness and attention, or (2) selective effects on certain brain structures and functions. In addition, individual differences in the effects have been reported.
The general explanation relies on the two-process model of sleep regulation. Cognitive impairments would be mediated through decreased alertness and attention through lapses, slowed responses, and wake-state instability. Attentional lapses, brief moments of inattentiveness, have been considered the main reason for the decrease in cognitive performance during sleep deprivation (on lapse hypothesis, eg, Williams et al 1959, see Dorrian et al 2005 ; Kjellberg 1977 ). The lapses are caused by microsleeps characterized by very short periods of sleep-like electro-encephalography (EEG) activity ( Priest et al 2001 ). Originally, it was thought that in between the lapses, cognitive performance almost remained intact, but the slowing of cognitive processing has also been observed independent of lapsing ( Kjellberg 1977 ; Dorrian et al 2005 ). According to these hypotheses, performance during SD would most likely deteriorate in long, simple, and monotonous tasks requiring reaction speed or vigilance. In addition to the lapses and response slowing, considerable fluctuations in alertness and effort have been observed during SD. According to the wake-state instability hypothesis, those fluctuations lead to variation in performance ( Doran et al 2001 ).
According to explanations on selective impact, SD interferes with the functioning of certain brain areas and thus impairs cognitive performance. This approach is also referred to as the ‘sleep-based neuropsychological perspective’ ( Babkoff et al 2005 ). Perhaps the most famous theory in this category is the prefrontal vulnerability hypothesis, first proposed by Horne (1993) . It suggests that SD especially impairs cognitive performances that depend on the prefrontal cortex. These include higher functions, such as language, executive functions, divergent thinking, and creativity. In order to show the SD effect, the tests should be complex, new, and interesting. A good performance would require cognitive flexibility and spontaneity. This theory also assumes that the deterioration of subjects’ performance in simple and long tasks is merely due to boredom ( Harrison and Horne 1998 ; Harrison and Horne 1999 ; Harrison and Horne 2000 ). The specific brain areas that are vulnerable to sleep loss have been explored using functional magnetic resonance imaging (fMRI) and positron emission tomography (PET). Those studies, however, have mainly measured working memory or other attentional functions with the type of tasks that are not traditionally emphasized in the prefrontal vulnerability hypothesis (for summary, see Chee et al 2006 ).
Individuals differ in terms of the length, timing, and structure of sleep. Therefore, it is logical to hypothesize that interindividual differences are also important in reaction to SD. Studies have consistently found that some people are more vulnerable to sleep loss than others (for review, see Van Dongen et al 2005 ). In reference to trait differential vulnerability to SD, Van Dongen et al (2005) have proposed the concept of the “trototype”, as compared to the terms “chronotype” and “somnotype”, which define interindividual differences in the timing of circadian rhythmicity and sleep duration. Since a comprehensive review of the interindividual differences in sleep and performance has been published recently ( Van Dongen et al 2005 ), we will focus here on the studies with group comparisons and just briefly address the trait-like vulnerability.
Acute total sleep deprivation
Attention and working memory.
The two most widely studied cognitive domains in SD research are attention and working memory, which in fact are interrelated. Working memory can be divided into four subsystems: phonological loop, visuospatial sketchpad, episodic buffer and central executive ( Baddeley and Hitch 1974 ; Baddeley 2000 ). The phonological loop is assumed to temporarily store verbal and acoustic information (echo memory); the sketchpad, to hold visuospatial information (iconic memory), and the episodic buffer to integrate information from several different sources. The central executive controls them all. Executive processes of working memory play a role in certain attentional functions, such as sustained attention ( Baddeley et al 1999 ), which is referred to here as vigilance. Both attention and working memory are linked to the functioning of frontal lobes (for a review, see Naghavi and Nyberg 2005 ). Since the frontal brain areas are vulnerable to SD ( Harrison et al 2000 ; Thomas et al 2000 ), it can be hypothesized that both attention and working memory are impaired during prolonged wakefulness.
The decrease in attention and working memory due to SD is well established. Vigilance is especially impaired, but a decline is also observed in several other attentional tasks ( Table 1 ). These include measures of auditory and visuo-spatial attention, serial addition and subtraction tasks, and different reaction time tasks ( Table 1 ). The most frequently used task is the psychomotor vigilance test (PVT, lasts usually 10 min) ( Dinges and Powell 1985 ), which is sensitive to sleep loss effects and provides information about both reaction speed and lapses. In working memory, the tests have varied from n-back style tasks with different demand levels to choice-reaction time tasks with a working memory component ( Table 1 ). However, some studies have also failed to find any effect. After one night of SD, no difference was observed between deprived and non-deprived subjects in simple reaction time, vigilance, or selective attention tasks in one study ( Forest and Godbout 2000 ). Performance on the Wisconsin Card Sorting Test, a measure of frontal lobe function, also remained even ( Binks et al 1999 ; Forest and Godbout 2000 ). These results may be partly biased because of small sample sizes, inadequate control of the subjects’ sleep history or the use of stimulants before the study.
Cognitive tests in which deterioration of performance has been reported during acute total sleep deprivation
Abbreviations: SD, sleep deprivation; WAIS, Wechsler Adult Intelligence Scale; WAIS-R, Wechsler Adult Intelligence Scale-Revised.
Outcomes are inconsistent in various dual tasks used for measuring divided attention. Sleep deprivation of 24 h impaired performance in one study ( Wright and Badia 1999 ), whereas in two others, performance was maintained after 25–35 h of SD ( Drummond et al 2001 ; Alhola et al 2005 ). The divergent findings in these studies may be explained by the uneven loads between different subtests as well as by uncontrolled practice effect. Although dividing attention between different tasks puts high demands on cognitive capacity, subjects often attempt to reduce the load by automating some easier procedures of a dual or multitask. In the study by Wright and Badia (1999) , the test was not described; in the study by Alhola et al (2005) , subjects had to count backwards and carry out a visual search task simultaneously, and in the study by Drummond et al (2001) subjects had to memorize words and complete a serial subtraction task sequentially. In addition, differences in essential study elements, like the age and gender of participants, as well as the duration of SD, further complicate comparison of the results.
In the tasks measuring attention or working memory, two aspects of performance are important: speed and accuracy. In practice, people can switch their emphasis between the two with attentional focusing ( Rinkenauer et al 2004 ). Oftentimes, concentrating on improving one aspect leads to the deterioration of the other. This is called the speed/accuracy trade-off phenomenon. Some SD studies have found impairment only in performance speed, whereas accuracy has remained intact ( De Gennaro et al 2001 ; Chee and Choo 2004 ). In others, the results are the opposite ( Kim et al 2001 ; Gosselin et al 2005 ). De Gennaro et al (2001) proposed that in self-paced tasks, there is likely to be a stronger negative impact on speed, while accuracy remains intact. In experimenter-paced tasks, the effect would be the opposite. However, many studies show detrimental effect on both speed and accuracy (eg, Smith et al 2002 ; Jennings et al 2003 ; Chee and Choo 2004 ; Habeck et al 2004 ; Choo et al 2005 ). The speed/accuracy trade-off phenomenon is moderately affected by gender, age, and individual differences in response style ( Blatter et al 2006 ; Karakorpi et al 2006 ), which could be a reason for inconsistencies in the SD results. It has been argued that low signal rates increase fatigue during performance in SD studies and that subjects may even fall asleep during the test ( Dorrian et al 2005 ). Therefore, tasks with different signal loads may produce different results in terms of performance speed and accuracy.
Long-term memory
Long-term memory can be divided between declarative and non-declarative (procedural) memory. Declarative memory is explicit and limited, whereas non-declarative memory is implicit and has a practically unlimited capacity. Declarative memory includes semantic memory, which consists of knowledge about the world, and episodic memory, which holds autobiographical information. The contents of declarative memory can be stored in visual or verbal forms and they can be voluntarily recalled. Non-declarative or procedural memory includes the information needed in everyday functioning and behavior, eg, motor and perceptual skills, conditioned functions and priming. In previous studies, long-term memory has been measured with a variety of tasks, and the results are somewhat inconsistent.
In verbal episodic memory, SD of 35 h impaired free recall, but not recognition ( Drummond et al 2000 ). The opposite results were obtained with one night of SD ( Forest and Godbout 2000 ). The groups in both studies were quite small (in Drummond’s study, N = 13; in Forest and Godbout’s study, experimental group = 9, control group = 9), which offers a possible explanation for the variation in results. In addition, Drummond et al (2000) used a within-subject design, whereas Forest and Godbout (2000) had a between-subject design. In visual memory, recognition was similar in the experimental and control groups when the measurement was taken once after 36 h SD ( Harrison and Horne 2000 ), whereas the practice effect in visual recall was postponed by SD in a study with three measurements (baseline, 25 h SD, recovery; Alhola et al 2005 ). Performance was impaired in probed forced memory recall ( Wright and Badia 1999 ), and memory search ( McCarthy and Waters 1997 ), but no effect was found in episodic memory ( Nilsson et al 2005 ), implicit memory, prose recall, crystallized semantic memory, procedural memory, or face memory ( Quigley et al 2000 ). In the studies failing to find an effect, however, the subjects spent only the SD night under controlled conditions ( Quigley et al 2000 ; Nilsson et al 2005 ).
Free recall and recognition are both episodic memory functions which seem to be affected differently by SD. Temporal memory for faces (recall) deteriorated during 36 h of SD, although in the same study, face recognition remained intact ( Harrison and Horne 2000 ). In verbal memory, the same pattern was observed ( Drummond et al 2000 ). One explanation may be different neural bases, which supports the prefrontal vulnerability hypothesis. Episodic memory is strongly associated with the functioning of the medial temporal lobes ( Scoville and Milner 2000 ), but during free recall in a rested state, even stronger brain activation is found in the prefrontal cortex ( Hwang and Golby 2006 ). It is unclear whether this prefrontal activation reflects episodic memory function, the organization of information in working memory, or the executive control of attention and memory. Recognition, instead, presumably relies on the thalamus in addition to medial temporal lobes ( Hwang and Golby 2006 ). Since SD especially disturbs the functioning of frontal brain areas ( Drummond et al 1999 ; Thomas et al 2000 ), it is not surprising that free recall is more affected than recognition.
Although the prefrontal cortex vulnerability hypothesis has received wide support in the field of SD research, other brain areas are also involved. For instance, the exact role of the thalamus remains unknown. Some studies measuring attention or working memory have noted an increase in thalamic activation during SD (eg, Portas et al 1998 ; Chee and Choo 2004 ; Habeck et al 2004 ; Choo et al 2005 ). This may reflect an increase in phasic arousal or an attempt to compensate attentional performance during a demanding condition of low arousal caused by SD ( Coull et al 2004 ). In other cognitive tasks such as verbal memory ( Drummond and Brown 2001 ) or logical reasoning ( Drummond et al 2004 ), no increase in thalamic activation was found despite the fact that behavioral deterioration occurred. This implies that thalamic activation during SD is mainly related to some attentional function or compensation, providing further support for the hypothesis that “prefrontal dependent” recall is more affected by SD than “thalamus dependent” recognition. However, it is possible that the brain activation patterns during SD reflect something more than merely different cognitive domains. Harrison and Horne (2000) stated that their results may also reflect the difficulty of the task assigned to subjects.
Other cognitive functions
Sleep deprivation impairs visuomotor performance, which is measured with tasks of digit symbol substitution, letter cancellation, trail-making or maze tracing ( Table 1 ). It is believed that visual tasks would be especially vulnerable to sleep loss because iconic memory has short duration and limited capacity ( Raidy and Scharff 2005 ). Another suggestion is that SD impedes engagement of spatial attention, which can be observed as impairments in saccadic eye movements ( Bocca and Denise 2006 ). Decreased oculomotor functioning is associated with impaired visual performance ( De Gennaro et al 2001 ) and sleepiness (eg, De Gennaro et al 2001 ; Zils et al 2005 ). However, further research is needed to confirm this explanation, since not all studies have found oculomotor impairment with cognitive performance decrements ( Quigley et al 2000 ).
Reasoning ability during SD has for the most part been measured with Baddeley’s logical reasoning task or its modified versions. Again the results are inconsistent (deteriorated performance was reported by Blagrove et al 1995 ; McCarthy and Waters 1997 ; Monk and Carrier 1997 , and Harrison and Horne 1999 ; no effects were noted by Linde and Bergstrom 1992 ; Quigley et al 2000 , or Drummond et al 2004 ). The studies reporting no effect have mainly used SD of ca. 24 h ( Linde and Bergström 1992 ; Quigley et al 2000 ), whereas in the studies showing an adverse effect, the SD period has been longer (36 h). Thus reasoning ability seems to be maintained during short-term SD. However, choosing divergent study designs may result in different outcomes. Monk and Carrier (1997) repeated the cognitive test every 2 h and found deterioration after as little as 16 h of SD. In the studies with zero-results, cognitive tests were carried out in the morning ( Linde and Bergström 1992 ; Quigley et al 2000 ) or the practice effect was not adequately controlled ( Drummond et al 2004 ). In the studies with longer SD, the tests have been conducted either in the late afternoon ( McCarthy and Waters 1997 ; Harrison and Horne 1999 ) or have been repeated several times ( Blagrove et al 1995 ; Monk and Carrier 1997 ). Therefore, the different results may reflect the effect of circadian rhythm on alertness and cognitive performance. In the morning or before noon, the circadian process reaches its peak, inducing greater alertness, whereas the timing of the circadian nadir coincides with the late afternoon testing (see Achermann 2004 ).
In addition to the cognitive domains already introduced, total SD affects several other cognitive processes as well. It increases rigid thinking, perseveration errors, and difficulties in utilizing new information in complex tasks requiring innovative decision-making ( Harrison and Horne 1999 ). Deterioration in decision-making also appears as more variable performance and applied strategies ( Linde et al 1999 ), as well as more risky behavior ( Killgore et al 2006 ). Several other tasks have been used in the sleep deprivation studies ( Table 1 ). For example, motor function, rhythm, receptive and expressive speech, and memory measured with the Luria-Nebraska Neuropsychological Battery deteriorated after one night of SD, whereas tactile function, reading, writing, arithmetic and intellectual processes remain intact ( Kim et al 2001 ).
The adverse effects of total SD shown in experimental designs have also been confirmed in real-life settings, mainly among health care workers, professional drivers and military personnel ( Samkoff and Jacques 1991 ; Otmani et al 2005 ; Philibert 2005 ; Russo et al 2005 ). Performance of residents in routine practice and repetitive tasks requiring vigilance becomes more error-prone when wakefulness is prolonged (for a review, see Samkoff and Jacques 1991 ). However, in new situations or emergencies, the residents seem to be able to mobilize additional energy sources to compensate for the effects of tiredness. More recent meta-analysis shows that SD of less than 30 h causes a significant decrease in both the clinical and overall performance of both residents and non-physicians ( Philibert 2005 ).
What role does motivation play in cognitive performance? Can high motivation reverse the adverse effect of SD? Does poor motivation further deteriorate performance? According to a commonly held opinion, high motivation compensates for a decrease in performance, but only a few attempts have been made to confirm this theory. Estimating the compensatory effect of motivation in performance during SD is generally difficult, because persons participating in research protocols, especially in SD studies, usually have high initial motivation. The concept of motivation is closely linked to the “attentional effort” that is considered a cognitive incentive (for a review, see Sarter et al 2006 ). According to Sarter et al (2006) , “increases in attentional effort do not represent primarily a function of task demands but of subjects’ motivation to perform.” Furthermore, attentional effort is a function of explicit and implicit motivational forces and may be increased especially when the subjects are motivated or when they detect signals of performance decrements ( Sarter et al 2006 ).
Harrison and Horne (1998 , 1999) suggest that the deterioration of cognitive performance during SD could be due to boredom and lack of motivation caused by repeated tasks, especially if the tests are simple and monotonous. They used short, novel, and interesting tasks to abolish this motivational gap, yet still noted that SD impaired performance. In contrast, other researchers suggest that sleep-deprived subjects could maintain performance in short tasks by being able to temporarily increase their attentional effort. When a task is longer, performance deteriorates as a function of time. A meta-analysis by Pilcher and Huffcutt (1996) provides support for that: total SD of less than 45 h deteriorated performance more severely in complex tasks with a long duration than in simple and short tasks. Based on this, it is probably necessary to make a distinction between mere attentional effort and more general motivation. Although attentional effort reflects motivational aspects in performance, motivation in a broader sense can be considered a long-term process such as achieving a previously set goal, eg, completing a study protocol. If one has already invested a great deal of time and effort in the participation, motivation to follow through may be increased.
Different aspects of motivation were investigated in a study with 72 h SD, where the subjects evaluated both motivation to perform the tasks and motivation to carry out leisure activities ( Mikulincer et al 1989 ). Cognitive tasks were repeated every two hours. Performance motivation decreased only during the second night of SD, whereas leisure motivation decreased from the second day until the end of the study on the third day. The authors concluded that the subjects were more motivated to complete experimental testing than to enjoy leisure activities because by performing the tasks, they could advance the completion of the study. The researchers suggested that the increased motivation towards the tasks on the third day reflected the “end spurt effect” caused by the anticipation of sleep.
Providing the subjects with feedback on their performance or rewarding them for effort or good performance is shown to help maintain performance both in normal, non-deprived conditions ( Tomporowski and Tinsley 1996 ) and during SD ( Horne and Pettitt 1985 ; Steyvers 1987 ; Steyvers and Gaillard 1993 ). In a large study with 61 subjects (experimental group = 29), with SD of 34–36 h, and with a comprehensive test battery, the subjects were continuously encouraged and provided with 2–3 minute breaks between the tests ( Binks et al 1999 ). Furthermore, they were told they would receive a monetary award for completing all tests with “honest effort”. As result, no deteriorating effect on cognitive performance was found. Unfortunately, a non-motivated control group was not included and thus the effect of motivation remained uncertain. In general, since this issue has not been addressed sufficiently, it is difficult to specify the role of motivation in performance. It seems that motivation affects performance, but it also appears that SD can lead to a loss of motivation.
Self-evaluation of cognitive performance
It has been suggested that the self-evaluation of cognitive performance is impaired by SD. During 36 h SD, the subjects became more confident that their answers were correct as the wakefulness continued ( Harrison and Horne 2000 ). Confidence was even stronger when the answer was actually wrong. In another study, performance was similar between sleep-deprived and control groups in several attentional assessments, but the deprived subjects evaluated their performance as moderately impaired ( Binks et al 1999 ). The controls considered that their performance was high.
The ability to evaluate one’s own cognitive performance depends on age and on the study design. Young people seem to underestimate the effect of SD, whereas older people seem to overestimate it. In a simple reaction time task, both young (aged 20–25 years) and aging (aged 52–63 years) subjects considered that their performance had deteriorated after 24 h SD, although performance was actually impaired only in young subjects ( Philip et al 2004 ). When it comes to the study design and methodology, the way in which the self-evaluation is done may affect the outcome. The answers possibly reflect presuppositions of the subjects or their desire to please the researcher. The repetition of tasks is also essential. Evaluation ability is poor in studies with one measurement only ( Binks et al 1999 ; Harrison and Horne 2000 ; Philip et al 2004 ), whereas in repeated measures, the subjects are shown to be able to assess their performance quite reliably during 60–64 h SD and recovery ( Baranski et al 1994 ; Baranski and Pigeau 1997 ). Thus, self-evaluation is likely to be more accurate when subjects can compare their performance with baseline.
Chronic partial sleep restriction
Although chronic partial sleep restriction is common in everyday life and even more prevalent than total SD, surprisingly few studies have evaluated its effects on cognitive performance. Even fewer studies have compared the effects of acute total sleep deprivation and chronic partial sleep restriction. Belenky and co-workers (2003) evaluated the effect of partial sleep restriction in a laboratory setting in groups which were allowed to spend 3, 5 or 7 h in bed daily for seven consecutive days. The control group spent 9 h in bed. In the 3 h group, both speed and accuracy in the PVT deteriorated almost linearly as the sleep restriction continued. In this group, performance was clearly the worst. In the 5- and 7 h groups, performance speed deteriorated after the first two restriction nights, but then remained stable (though impaired) during the rest of the sleep restriction from the third night onwards. Impairment was greater in the 5- than 7 h group. Accuracy followed the same pattern in the 7 h group, but further declined in the 5 h group as the study went on. The control group’s performance did not change during the study. Intriguingly, a highly similar pattern was observed in another study with the same task when sleep was restricted by 33% of the subject’s habitual nightly sleep, which resulted in 5 h of sleep per night on average ( Dinges et al 1997 ). Both speed and accuracy were impaired at the beginning of the sleep restriction period followed by a plateau and finally, another drop after the seventh night of deprivation. However, no change was found in probed recall memory or serial addition tests, probably because of the practice effect and short duration of the tests (serial addition test: 1 min).
It is difficult to compare the effects of total and partial SD based on existing literature due to large variation in methodologies, including the length of SD or the type of cognitive measures. The only study that has compared total and partial SD found that after controlling learning effects, cognitive performance declined almost linearly in the course of the study in all four experimental groups ( Van Dongen et al 2003a ): one group was exposed to 3 nights total SD, and in other experimental groups, time in bed was restricted to 4 or 6 h for 14 consecutive days. The control group was allowed 8 h in bed for 14 days. Impairment in psychomotor vigilance test and digit symbol substitution task for the 4 h group after 14 days was equal to that of the total SD group after 2 nights. Deterioration in the serial addition/subtraction task for the 4 h group was similar to that of the total SD group after 1 night. The effect of 6 h restricted sleep corresponded to 1 night of total SD in psychomotor vigilance and digit symbol. Performance remained unaffected in the control group.
According to the well-controlled studies ( Dinges et al 1997 ; Belenky et al 2003 ; Van Dongen et al 2003a ), the less sleep obtained due to sleep restriction, the more cognitive performance is impaired. Otherwise, it is difficult to draw conclusions about the effects of chronic sleep restriction because of methodological problems in the previous studies. Blagrove et al (1995) compared subjects that slept at home either 5 h or 8 h per night for 4 weeks and found no effect in a short task of logical reasoning (duration 5 min). The statistical analyses were compromised by the small sample size (6 subjects in the experimental group and only 4 subjects in the control group). In another protocol, they also carried out auditory vigilance test, two column addition, finding embedded figures, and logical reasoning (10 min) tasks, and again no effect was observed with groups of 6–8 subjects having 4, 5 or 8 h sleep per night for 7, 19 or 40 weeks respectively ( Blagrove et al 1995 ). Casement et al (2006) reported no change in working memory and motor speed in the group whose sleep was restricted to 4 h per night for 9 nights. In the control group, performance improved. The study was carried out in a controlled clinical environment, but only one short test session per day was included, which means that subjects may have been able to temporarily increase their effort and thus maintain their performance. Furthermore, the results were confounded by the practice effect. In other sleep restriction studies, SD cannot be considered chronic, since the length of the restriction has been 1–3 nights ( Stenuit and Kerkhofs 2005 ; Swann et al 2006 ; Versace et al 2006 ).
Since chronic partial SD mimics every day life situations more than acute total SD, additional studies on how it affects cognitive performance are warranted. In addition, the tasks used in previous studies have been quite short and simple, and trials with more demanding cognitive tasks are required. The effects of sleep restriction have also been addressed by drive simulation studies, which are interesting and practical designs. Just one night of restricted sleep (4 h) increased right edge-line crossings in a motorway drive simulation of 90 minutes ( Otmani et al 2005 ). However, neither the drivers’ position in the lane nor the amplitude and frequency of steering wheel movements were affected. One sleep-restricted night did not increase the probability of a crash, but after five nights of partial SD, the quantity of accidents increased ( Thorne et al 1999 ).
Cognitive recovering from sleep deprivation
The recovery processes of cognitive performance after sleep loss are still obscure. In many SD studies, the recovery period has either not been included in the protocol or was not reported. Recovery sleep is distinct from normal sleep. Sleep latency is shorter, sleep efficiency is higher, the amounts of SWS and REM-sleep are increased and percentages of stage 1 sleep and awake are decreased ( Armitage et al 2001 ; Kilduff et al 2005 ). The characteristics of recovery sleep may also depend on circumstances and some differences seem to come with eg, aging ( Kalleinen et al 2006 ). Evidence suggests that one sleep period (at least eight hours) can reverse the adverse effects of total SD on cognition ( Brendel et al 1990 ; Corsi-Cabrera et al 2003 ; Adam et al 2006 ; Drummond et al 2006 ; Kendall et al 2006 ). The tasks have been mainly simple attentional tasks; for example, the PVT used by Adam et al (2006) has been proven to have practically no learning curve and little if any correlation with aptitude ( Durmer and Dinges 2005 ). Thus, it is likely that the improvement was mostly caused by the recovery process and not just the practice effect.
After chronic partial sleep restriction, the recovery process of cognitive functioning seems to take longer than after acute total SD. Performance in the PVT was not restored after one 10 h recovery night, but approached the baseline level after two 10 h nights in a study with seven consecutive sleep restriction nights with 5 h sleep/night ( Dinges et al 1997 ). Using the same test, three 8 h recovery nights were not enough to restore performance after one week of sleep restriction even in the group that spent 7 h time in bed (the study is explained in greater detail in paragraph 1 of “Partial sleep restriction”, Belenky et al 2003 ). The group that spent 3 h in bed showed the greatest decline as well as the greatest recovery, although it did not reach baseline level again. In the 5 h group, a similar deterioration-recovery curve was observed, although it was not as steep. Those authors concluded that during mild and moderate chronic partial SD, the brain adapted to a stressful condition to maintain performance, yet at a reduced level. This adaptation process was obviously so demanding that it postponed the restoration of normal functioning. According to their results, it could be further interpreted that when sleep restriction was severe, no such adaptation occurred, which in turn allowed for greater recovery. However, these results may be biased because of poor statistical sensitivity in multiple comparisons. They have also been criticized by eg, Van Dongen et al (2004) , who pointed out that another confounding factor may have been considerable interindividual differences in recovery rates. Since interindividual differences have been observed in response to SD, it is likely – although not yet adequately verified – that those individual traits also affect the recuperation.
Sleep deprivation in different populations
Sleep structure changes with aging. Slow wave sleep and sleep efficiency decrease, and alterations in the circadian rhythm occur (for reviews, see Dzaja et al 2005 ; Gaudreau et al 2005 ). Sleep complaints also become more frequent ( Leger et al 2000 ). Yet, during prolonged wakefulness, cognitive performance seems to be maintained better in aging people than in younger ones ( Bonnet and Rosa 1987 ; Smulders et al 1997 ; Philip et al 2004 ; Stenuit and Kerkhofs 2005 ). Total SD of 24 h deteriorated vigilance in young subjects (20–25 years), whereas performance in aging subjects (52–63 years) remained unaffected ( Philip et al 2004 ). Similarly, during three consecutive nights of partial SD (4 h in bed) performance in psychomotor vigilance task declined more in young subjects (20–30 years) than in aging ones (55–65 years, Stenuit and Kerkhofs 2005 ). In visual episodic memory, visuomotor performance and divided attention, aging subjects (58–72 years) were able to maintain their performance after 25 h of SD and showed improvement only after a recovery night ( Alhola et al 2005 ). However, no comparison with young subjects was made in that study.
Sleep deprivation deteriorates accuracy of performance, especially in young subjects ( Brendel et al 1990 ; Smulders et al 1997 ; Adam et al 2006 ; Karakorpi et al 2006 ). Regarding performance speed, however, results have been inconsistent and the performance of aging subjects has declined more, less, or equally compared to that of younger people. In simple and two-choice reaction time tasks as well as in a vigilance task, reaction speed was impaired in aging subjects (59–72 years) during 40 h SD, whereas young subjects (20–26 years) kept up their speed ( Karakorpi et al 2006 ). These results followed the speed/accuracy trade-off phenomenon so that aging subjects maintained accuracy at the expense of speed and the younger ones did the opposite. In contrast, two other studies found that young subjects were slower than aging subjects ( Brendel et al 1990 ; Adam et al 2006 ). During 24 h wakefulness, performance speed in a vigilance task was impaired in both 20- and 80-year-olds, but more so in the young subjects ( Brendel et al 1990 ). This was confirmed in another study with 40 h SD ( Adam et al 2006 ). When measuring reaction speed in three different choice-reaction time tasks, performance deteriorated similarly in young (18–24 years) and aging (62–73 years) subjects after 28 h total SD ( Smulders et al 1997 ).
Even though there is some evidence that older subjects tolerate SD better than young subjects, it is difficult to determine the age effect during SD with precision. However, because of age-related changes in many aspects of sleep and wakefulness, it is plausible that aging influences reactions to SD. As suggested previously, the weaker SD effect in aging may be due to attenuation of the circadian amplitude, which is reflected in the performance curve in vigilance tasks ( Blatter et al 2006 ). Also, changes in the homeostatic process may play a role. During wakefulness, the accumulation of sleep pressure seems to be reduced in aging ( Murillo-Rodriguez et al 2004 ), which could leave older subjects more alert. There is also evidence that aging subjects recover faster from SD than young subjects in terms of physiological sleep ( Bonnet and Rosa 1987 ; Brendel et al 1990 ). This faster recovery in sleep state may also mean better restoration of cognitive performance ( Bonnet and Rosa 1987 ; Brendel et al 1990 ). However, more research is necessary to confirm these hypotheses.
The age effect found in previous studies could also be explained by methodological factors, such as inadequate control of the baseline conditions. Younger subjects are usually more chronically sleep deprived ( National Sleep Foundation 2002 ) due to several reasons, such as studying, career building or raising children. Chronic sleep restriction may cause long-term changes in brain functions that are not reversible during short adaptation and baseline periods in sleep laboratory studies. Even though subjects of certain studies were instructed to maintain a regular 8 h sleep schedule for 3–5 days, this may not be enough to erase the previous “sleep debt” ( Brendel et al 1990 ; Philip et al 2004 ; Adam et al 2006 ). Furthermore, in the long run, people tend to get used to experiencing sleepiness ( Van Dongen et al 2003a ) and thus may not even recognize being chronically sleep deprived. Perhaps aging people also have more experience that helps them to cope with the challenges posed by SD. Nevertheless, based on the available studies, it is impossible to distinguish the factors behind the age effect.
There are dissimilarities between genders in sleep structure measured with polysomnography (for a review, see Manber and Armitage 1999 ). Furthermore, women of all ages report more sleeping problems than men ( Leger et al 2000 ). Sex hormones affect sleep through several mechanisms, both genomic and nongenomic, including neurochemical and vascular mechanisms (for a review, see Dzaja et al 2005 ). This ensures instant and short-term effects as well as long-term ones.
It is possible that physiological responses to SD are not equal among men and women. During SD of 38 h, EEG showed more sleep activity in men than in women during waking rest and cognitive performance ( Corsi-Cabrera et al 2003 ). Presumably, therefore, one recovery night of nine hours would be enough to restore waking EEG activity in men, but not in women. Only a few studies have examined gender differences in cognitive performance during SD. In a vigilance task, performance was more impaired in men but returned to the baseline level in both men and women after recovery sleep ( Corsi-Cabrera et al 2003 ). In another study, women performed better than men in verbal and in visuo-constructive tasks during 35 h SD ( Binks et al 1999 ). No gender differences were observed in word fluency, maintenance or suppression of attention, auditory attention or cognitive flexibility. In that study, however, only one point of measurement was included, and so the difference in performance could be caused by SD or initial distinctions between the gender groups.
Few attempts have been made to evaluate the effect of sex hormones on coping with SD. It has been suggested that hormone therapy, which is widely used for women during their menopausal transition to help alleviate climacteric symptoms, attenuates physiological stress response ( Lindheim et al 1992 ). However, after 25 h of total SD, no difference was observed between hormone therapy users and nonusers in visual episodic memory, visuomotor performance, verbal attention and shared attention ( Alhola et al 2005 ). In addition, during 40 h of SD, hormone therapy did not produce any advantage in reaction time or vigilance tasks ( Karakorpi et al 2006 ).
The previous studies suggest that women cope with continuous wakefulness better than men. According to evolution, the demands of child nurturing and rearing in women would support this hypothesis ( Corsi-Cabrera et al 2003 ), but that certainly does not constitute a comprehensive explanation today. Gender differences during SD could be due to either physiological or social factors. There are differences in the brain structure and functioning of men and women ( Ragland et al 2000 ; Cowell et al 2007 ). These can be seen in cognitive performance in normal, non-deprived conditions: men typically have better spatial abilities and mental rotation, and higher visuo-constructive performance, whereas women perform better in visuomotor speed and some verbal functions, especially verbal fluency (for a review, see Kimura 1996 ). Men and women also exhibit behavioral and lifestyle differences, which are mainly due to socialization and gender roles ( Eagly and Wood 1999 ). Current literature, however, provides only minimal evidence of differential effects during SD, and does not resolve the issue of sexual dimorphism in coping with SD.
Interindividual differences
Several studies provide evidence that during total SD, performance becomes more variable as assessed from the within-subject point of view (eg, Smith et al 2002 ; Habeck et al 2004 ; Choo et al 2005 ). This is considered to reflect the wake-state instability caused by prolonged wakefulness. However, Doran et al (2001) were probably the first to also examine between-subjects variability, which they found to increase in PVT as wakefulness was extended to 88 hours. They suggested that some people are more vulnerable to the effects of sleep loss than others, which could probably explain the lack of significant results in some group comparisons. These differences between subjects could have arguably been caused by differences in sleep history, but the sleep patterns for the preceding week were controlled with sleep diaries, actigraph, and calls to the time-stamped voice recorder.
The interindividual variability has been further examined with a thorough protocol where a three night study (baseline, 36 h SD and recovery) was carried out three times ( Van Dongen et al 2004 ). Sleep history was manipulated by instructing subjects to stay in bed for either 6 or 12 h per night for one week before the study. The 12 h procedure was repeated and the order of the conditions was counterbalanced. The cognitive test selection included serial addition/subtraction task, digit symbol, critical tracking, word detection, repeated acquisition of response sequences, and PVT. The authors concluded that interindividual differences were systematic and independent from sleep history. The trait-like differential vulnerability to sleep loss has received support from an fMRI study attempting to reveal the neural basis for the interindividual differences ( Chuah et al 2006 ). They used a go/no-go task to measure response inhibition after 24 h of sleep deprivation. The results indicated that the subjects less vulnerable to SD had lower prefrontal cortex activation at the rested wakefulness than the more vulnerable subjects. During SD, activation increased temporarily in the prefrontal cortex and in some other areas only in the less vulnerable subjects. Since interindividual differences have also been found in other sleep-related variables, such as duration, timing, and quality of sleep, sleepiness, and circadian phase ( Van Dongen 1998 ; Van Dongen et al 2005 ), it is plausible that the tolerance to SD may also vary. Nevertheless, more studies are needed for further support.
Methodological issues and common biases
Although the adverse effects of SD on cognitive performance are quite well established, some studies have failed to detect any deterioration. Inadequate descriptions of study protocols or subject characteristics in some studies make it difficult to interpret the neutral results. However, it is likely that such results are due to methodological shortcomings, such as insensitive cognitive measures, failure to control the practice effect or other confounding factors, like individual sleep history or napping during the study. Also, if the task is carried out only once during the SD period, the results may be influenced by circadian rhythm.
Sleep deprivation studies are laborious and expensive to carry out, which may lead to compromises in the study design: for example, a small sample size can reduce the statistical power of the study, but a larger population may come at the expense of other methodological issues, such as a reduction in the cognitive test selection or in the number of nights spent in the sleep laboratory. Comparison of the results is also complicated because the length of sleep restriction varies and the studies are designed either within- or between-subjects.
Sleeping in unfamiliar surroundings may impair sleep quality. An adaptation night at the sleep laboratory is used to minimize this first night effect. However, in several studies, this has been neglected and the SD period has been preceded by a “normal” night at home (eg, Harrison and Horne 2000 ; Jennings et al 2003 ; Choo et al 2005 ). Although sleeping at home certainly reflects a subject’s reality more accurately, it does not allow for precise control and information of sleeping conditions. Adding a portable recording, such as an actigraph, provides objective information about eg, bedtime and resting periods. In some studies, the first night in the sleep laboratory has been the baseline (eg, Drummond et al 2000 ; Forest and Godbout 2000 ; De Gennaro et al 2001 ; Drummond et al 2001 ), whereas others have included one adaptation night (eg, Casagrande et al 1997 ; Alhola et al 2005 ). Yet, it may be questionable to use data from the second night as the baseline because sleep quality can be better than normal due to the rebound from the first night. Accordingly, only data from the third night should be accepted, which has been the case in a few studies ( Thomas et al 2000 ; Van Dongen et al 2003a ). This, however, makes the procedure very hard. Furthermore, study protocols can be improved by adding an ambulatory EEG recording to confirm the wakefulness of the subjects during the study.
In sleep studies, a common pitfall is recruitment methods. Enrolment via advertisements or from sleep clinics favors the selection of subjects with sleeping problems or concerns about their cognitive performance. Thus, strict exclusion criteria regarding physical or mental diseases or sleeping problems are essential. Further, sleeping habits should be controlled to make sure that the subjects are not initially sleep deprived. For this, use of a sleep diary for eg, 1–3 weeks before the experiment (eg, De Gennaro et al 2001 ; Habeck et al 2004 ; Alhola et al 2005 ) or an actigraph is applicable ( Harrison and Horne 1999 ; Thomas et al 2000 ).
The use of medication or stimulants, such as caffeine, alcohol or tobacco, is often prohibited before the experiment (eg, Thomas et al 2000 ; Van Dongen et al 2003a ; Habeck et al 2004 ; Alhola et al 2005 ; Choo et al 2005 ). In some studies, the subjects have been required to refrain from these substances only 24 h before the study ( Habeck et al 2004 ; Choo et al 2005 ), which may increase withdrawal symptoms and dropping out of the study. Thus a longer abstinence, eg, 1–2 weeks, is more appropriate ( Van Dongen et al 2003a ; Alhola et al 2005 ).
A variety of cognitive tests, from simple reaction time measures to complex decision-making tasks requiring creativity and reasoning, have been used to evaluate the effect of SD on cognition. The greatest problem in repeated cognitive testing is the practice effect, which easily conceals any adverse effects of SD. Therefore, careful control over learning is essential. Cognitive processes are also intertwined in several ways, which makes it difficult to specify exactly which cognitive functions are utilized in certain performances. Because attention is involved in performing any cognitive task, a decrease in other cognitive domains during SD may be mediated through impaired attention. In complex tasks, however, applying previous knowledge and use of strategies or creativity may be more essential. Some studies have concentrated on neural correlates of cognitive functioning during continuous wakefulness. Both fMRI ( Portas et al 1998 ; Drummond et al 2000 ; Drummond et al 2001 ; Chee and Choo 2004 ; Habeck et al 2004 ; Choo et al 2005 ) and PET have been used ( Thomas et al 2000 ). Although these trials yield interesting information about brain functioning, the use of imaging techniques limits the selection of cognitive tests that could be carried out at the same time.
Dorrian et al (2005) have compiled a list of criteria for neurocognitive tests that would be suitable for investigating sleep deprivation effects. The criteria include psychometric quality, ie, reliability and validity, but the tests should also reflect a fundamental aspect of waking neurocognitive functions and it should be possible to interpret them in a meaningful way. The tasks should be repeatable, independent of aptitude, and they should be short with a high signal load. These criteria are not met in some studies. Dorrian et al (2005) also argued that vigilance is the underlying factor through which the sleep deprivation effects are mediated in all other tasks. However, although attention is needed to perform any task to some extent, the hypothesis that sleep deprivation can have an independent effect on other cognitive functions such as memory cannot be ruled out. Nevertheless, when measuring other cognitive functions, the characteristics of the task should be considered carefully and, eg, for repeated measures of memory, parallel test versions should be used.
The negative effect of both acute total and chronic partial SD on attention and working memory is supported by existing literature. Total SD impairs a range of other cognitive functions as well. In partial SD, a more thorough evaluation of higher cognitive functions is needed. Furthermore, the effects of SD have not been thoroughly compared among some essential subpopulations.
Aging influences a person’s ability to cope with SD. Although in general the cognitive performance of aging people is often poorer than that of younger individuals, during SD performance in older subjects seems to deteriorate less. Based on the scarce evidence, it seems that in terms of cognitive performance, women may endure prolonged wakefulness better than men, whereas physiologically they recover slower. Tolerating SD can also depend on individual traits. However, mechanisms inducing differences between the young and aging and between men and women or different individuals are mostly unclear. Several reasons such as physiological mechanisms as well as social or environmental factors may be involved. In conclusion, there is great variation in SD studies in terms of both subject selections and methods, and this makes it difficult to compare the different studies. In the future, methodological issues should be considered more thoroughly.
- Achermann P. The two-process model of sleep regulation revisited. Aviat Space Environ Med. 2004; 75 :A37–43. [ PubMed ] [ Google Scholar ]
- Adam M, Retey JV, Khatami R, et al. Age-related changes in the time course of vigilant attention during 40 hours without sleep in men. Sleep. 2006; 29 :55–7. [ PubMed ] [ Google Scholar ]
- Akerstedt T, Folkard S. Validation of the S and C components of the three-process model of alertness regulation. Sleep. 1995; 18 :1–6. [ PubMed ] [ Google Scholar ]
- lhola P, Tallus M, Kylmälä M, et al. Sleep deprivation, cognitive performance, and hormone therapy in postmenopausal women. Menopause. 2005; 12 :149–55. [ PubMed ] [ Google Scholar ]
- Armitage R, Smith C, Thompson S, et al. Sex differences in slow-wave activity in response to sleep deprivation. Sleep Research Online. 2001; 4 (1):33–41. [ Google Scholar ]
- Babkoff H, Zukerman G, Fostick L, et al. Effect of the diurnal rhythm and 24 h of sleep deprivation on dichotic temporal order judgment. J Sleep Res. 2005; 14 :7–15. [ PubMed ] [ Google Scholar ]
- Baddeley AD, Hitch GJ. Working memory . Academic Press; 1974. pp. 47–89. [ Google Scholar ]
- Baddeley A. The episodic buffer: A new component of working memory? Trends Cogn Sci. 2000; 4 :417–23. [ PubMed ] [ Google Scholar ]
- Baddeley A, Cocchini G, Della Sala S, et al. Working memory and vigilance: Evidence from normal aging and alzheimer’s disease. Brain Cogn. 1999; 41 :87–108. [ PubMed ] [ Google Scholar ]
- Baranski JV, Pigeau RA. Self-monitoring cognitive performance during sleep deprivation: Effects of modafinil, d-amphetamine and placebo. J Sleep Res. 1997; 6 :84–91. [ PubMed ] [ Google Scholar ]
- Baranski JV, Pigeau RA, Angus RG. On the ability to self-monitor cognitive performance during sleep deprivation: A calibration study. J Sleep Res. 1994; 3 :36–44. [ PubMed ] [ Google Scholar ]
- Belenky G, Wesensten NJ, Thorne DR, et al. Patterns of performance degradation and restoration during sleep restriction and subsequent recovery: A sleep dose-response study. J Sleep Res. 2003; 12 :1–12. [ PubMed ] [ Google Scholar ]
- Binks PG, Waters WF, Hurry M. Short-term total sleep deprivations does not selectively impair higher cortical functioning. Sleep. 1999; 22 :328–34. [ PubMed ] [ Google Scholar ]
- Blagrove M, Alexander C, Horne JA. The effects of chronic sleep reduction on the performance of cognitive tasks sensitive to sleep deprivation. App Cogn Psych. 1995; 9 :21–40. [ Google Scholar ]
- Blatter K, Graw P, Munch M, et al. Gender and age differences in psychomotor vigilance performance under differential sleep pressure conditions. Behav Brain Res. 2006; 168 :312–7. [ PubMed ] [ Google Scholar ]
- Bocca ML, Denise P. Total sleep deprivation effect on disengagement of spatial attention as assessed by saccadic eye movements. Clin Neurophysiol. 2006; 117 :894–9. [ PubMed ] [ Google Scholar ]
- Bonnet MH, Rosa RR. Sleep and performance in young adults and older normals and insomniacs during acute sleep loss and recovery. Biol Psychol. 1987; 25 :153–72. [ PubMed ] [ Google Scholar ]
- Brendel DH, Reynolds CF, 3rd, Jennings JR, et al. Sleep stage physiology, mood, and vigilance responses to total sleep deprivation in healthy 80-year-olds and 20-year-olds. Psychophysiology. 1990; 27 :677–85. [ PubMed ] [ Google Scholar ]
- Carskadon MA, Dement WC. Normal human sleep: An overview. Philadelphia: Elsevier Saunders; 2005. pp. 13–23. [ Google Scholar ]
- Casagrande M, Violani C, Curcio G, et al. Assessing vigilance through a brief pencil and paper letter cancellation task (LCT): Effects of one night of sleep deprivation and of the time of day. Ergonomics. 1997; 40 :613–30. [ PubMed ] [ Google Scholar ]
- Casement MD, Broussard JL, Mullington JM, et al. The contribution of sleep to improvements in working memory scanning speed: A study of prolonged sleep restriction. Biol Psychol. 2006; 72 :208–12. [ PMC free article ] [ PubMed ] [ Google Scholar ]
- Chee MW, Choo WC. Functional imaging of working memory after 24 hr of total sleep deprivation. J Neurosci. 2004; 24 :4560–7. [ PMC free article ] [ PubMed ] [ Google Scholar ]
- Chee MW, Chuah LY, Venkatraman V, et al. Functional imaging of working memory following normal sleep and after 24 and 35 h of sleep deprivation: Correlations of fronto-parietal activation with performance. Neuroimage. 2006; 31 :419–28. [ PubMed ] [ Google Scholar ]
- Choo WC, Lee WW, Venkatraman V, et al. Dissociation of cortical regions modulated by both working memory load and sleep deprivation and by sleep deprivation alone. Neuroimage. 2005; 25 :579–87. [ PubMed ] [ Google Scholar ]
- Chuah YM, Venkatraman V, Dinges DF, et al. The neural basis of interindividual variability in inhibitory efficiency after sleep deprivation. J Neurosci. 2006; 26 :7156–62. [ PMC free article ] [ PubMed ] [ Google Scholar ]
- Corsi-Cabrera M, Sanchez AI, del-Rio-Portilla Y, et al. Effect of 38 h of total sleep deprivation on the waking EEG in women: Sex differences. Int J Psychophysiol. 2003; 50 :213–24. [ PubMed ] [ Google Scholar ]
- Coull JT, Jones MEP, Egan TD, et al. Attentional effects of nor-adrenaline vary with arousal level: Selective activation of thalamic pulvinar in humans. NeuroImage. 2004/5; 22 :315–22. [ PubMed ] [ Google Scholar ]
- Cowell PE, Sluming VA, Wilkinson ID, et al. Effects of sex and age on regional prefrontal brain volume in two human cohorts. Eur J Neurosci. 2007; 25 :307–18. [ PubMed ] [ Google Scholar ]
- De Gennaro L, Ferrara M, Curcio G, et al. Visual search performance across 40 h of continuous wakefulness: Measures of speed and accuracy and relation with oculomotor performance. Physiol Behav. 2001; 74 :197–204. [ PubMed ] [ Google Scholar ]
- Dinges DF, Douglas SD, Zaugg L, et al. Leukocytosis and natural killer cell function parallel neurobehavioral fatigue induced by 64 hours of sleep deprivation. J Clin Invest. 1994; 93 :1930–9. [ PMC free article ] [ PubMed ] [ Google Scholar ]
- Dinges DF, Pack F, Williams K, et al. Cumulative sleepiness, mood disturbance, and psychomotor vigilance performance decrements during a week of sleep restricted to 4–5 hours per night. Sleep. 1997; 20 :267–77. [ PubMed ] [ Google Scholar ]
- Dinges DF, Powell JW. Microcomputer analyses of performance on a portable, simple visual RT task during sustained operations. Behav Res Meth Instr Comp. 1985; 17 :652–5. [ Google Scholar ]
- Doran SM, Van Dongen HP, Dinges DF. Sustained attention performance during sleep deprivation: Evidence of state instability. Arch Ital Biol. 2001; 139 :253–67. [ PubMed ] [ Google Scholar ]
- Dorrian J, Rogers NL, Dinges DF. Psychomotor vigilance performance: Neurocognitive assay sensitive to sleep loss. New York: Marcel Dekker; 2005. pp. 39–70. [ Google Scholar ]
- Drummond SP, Brown GG. The effects of total sleep deprivation on cerebral responses to cognitive performance. Neuropsychopharmacology. 2001; 25 :S68–73. [ PubMed ] [ Google Scholar ]
- Drummond SP, Brown GG, Gillin JC, et al. Altered brain response to verbal learning following sleep deprivation. Nature. 2000; 403 :655–7. [ PubMed ] [ Google Scholar ]
- Drummond SP, Brown GG, Salamat JS, et al. Increasing task difficulty facilitates the cerebral compensatory response to total sleep deprivation. Sleep. 2004; 27 :445–51. [ PubMed ] [ Google Scholar ]
- Drummond SP, Brown GG, Stricker JL, et al. Sleep deprivation-induced reduction in cortical functional response to serial subtraction. Neuroreport. 1999; 10 :3745–8. [ PubMed ] [ Google Scholar ]
- Drummond SP, Gillin JC, Brown GG. Increased cerebral response during a divided attention task following sleep deprivation. J Sleep Res. 2001; 10 :85–92. [ PubMed ] [ Google Scholar ]
- Drummond SP, Paulus MP, Tapert SF. Effects of two nights sleep deprivation and two nights recovery sleep on response inhibition. J Sleep Res. 2006; 15 :261–5. [ PubMed ] [ Google Scholar ]
- Durmer JS, Dinges DF. Neurocognitive consequences of sleep deprivation. Semin Neurol. 2005; 25 :117–29. [ PubMed ] [ Google Scholar ]
- Dzaja A, Arber S, Hislop J, et al. Women’s sleep in health and disease. J Psychiatr Res. 2005; 39 :55–76. [ PubMed ] [ Google Scholar ]
- Eagly AH, Wood W. The Origins of Sex Differences in Human Behavior: Evolved Dispositions Versus Social Roles. Am Psychol. 1999; 54 :408–23. [ Google Scholar ]
- Forest G, Godbout R. Effects of sleep deprivation on performance and EEG spectral analysis in young adults. Brain Cogn. 2000; 43 :195–200. [ PubMed ] [ Google Scholar ]
- Frey DJ, Badia P, Wright KP., Jr Inter- and intra-individual variability in performance near the circadian nadir during sleep deprivation. J Sleep Res. 2004; 13 :305–15. [ PubMed ] [ Google Scholar ]
- Gaudreau H, Carrier J, Tchiteya B. Age and individual determinants of sleep loss effects. New York: Marcel Dekker; 2005. pp. 441–479. [ Google Scholar ]
- Gosselin A, De Koninck J, Campbell KB. Total sleep deprivation and novelty processing: Implications for frontal lobe functioning. Clin Neurophysiol. 2005; 116 :211–22. [ PubMed ] [ Google Scholar ]
- Graw P, Krauchi K, Knoblauch V, et al. Circadian and wake-dependent modulation of fastest and slowest reaction times during the psychomotor vigilance task. Physiol Behav. 2004; 80 :695–701. [ PubMed ] [ Google Scholar ]
- Habeck C, Rakitin BC, Moeller J, et al. An event-related fMRI study of the neurobehavioral impact of sleep deprivation on performance of a delayed-match-to-sample task. Brain Res Cogn Brain Res. 2004; 18 :306–21. [ PubMed ] [ Google Scholar ]
- Harrison Y, Espelid E. Loss of negative priming following sleep deprivation. Q J Exp Psychol A. 2004; 57 :437–46. [ PubMed ] [ Google Scholar ]
- Harrison Y, Horne JA. Sleep loss and temporal memory. Q J Exp Psychol A. 2000; 53 :271–9. [ PubMed ] [ Google Scholar ]
- Harrison Y, Horne JA. One night of sleep loss impairs innovative thinking and flexible decision making. Organ Behav Hum Decis Process. 1999; 78 :128–45. [ PubMed ] [ Google Scholar ]
- Harrison Y, Horne JA. Sleep loss impairs short and novel language tasks having a prefrontal focus. J Sleep Res. 1998; 7 :95–100. [ PubMed ] [ Google Scholar ]
- Harrison Y, Horne JA, Rothwell A. Prefrontal neuropsychological effects of sleep deprivation in young adults–a model for healthy aging? Sleep. 2000; 23 :1067–73. [ PubMed ] [ Google Scholar ]
- Heuer H, Klein W. One night of total sleep deprivation impairs implicit learning in the serial reaction task, but not the behavioral expression of knowledge. Neuropsychology. 2003; 17 :507–16. [ PubMed ] [ Google Scholar ]
- Heuer H, Kleinsorge T, Klein W, et al. Total sleep deprivation increases the costs of shifting between simple cognitive tasks. Acta Psychol (Amst) 2004; 117 :29–64. [ PubMed ] [ Google Scholar ]
- Heuer H, Kohlisch O, Klein W. The effects of total sleep deprivation on the generation of random sequences of key-presses, numbers and nouns. Q J Exp Psychol A. 2005; 58 :275–307. [ PubMed ] [ Google Scholar ]
- Heuer H, Spijkers W, Kiesswetter E, et al. Effects of sleep loss, time of day, and extended mental work on implicit and explicit learning of sequences. J Exp Psychol Appl. 1998; 4 :139–62. [ PubMed ] [ Google Scholar ]
- Horne JA. Human sleep, sleep loss and behaviour. implications for the prefrontal cortex and psychiatric disorder. Br J Psychiatry. 1993; 162 :413–9. [ PubMed ] [ Google Scholar ]
- Horne JA, Pettitt AN. High incentive effects on vigilance performance during 72 hours of total sleep deprivation. Acta Psychol (Amst) 1985; 58 :123–39. [ PubMed ] [ Google Scholar ]
- Hwang DY, Golby AJ. The brain basis for episodic memory: Insights from functional MRI, intracranial EEG, and patients with epilepsy. Epilepsy Behav. 2006; 8 :115–26. [ PubMed ] [ Google Scholar ]
- Jennings JR, Monk TH, van der Molen MW. Sleep deprivation influences some but not all processes of supervisory attention. Psychol Sci. 2003; 14 :473–9. [ PubMed ] [ Google Scholar ]
- Johnsen BH, Laberg JC, Eid J, et al. Dichotic listening and sleep deprivation: Vigilance effects. Scand J Psychol. 2002; 43 :413–17. [ PubMed ] [ Google Scholar ]
- Kalleinen N, Polo O, Himanen SL, et al. Sleep deprivation and hormone therapy in postmenopausal women. Sleep Med. 2006; 7 :436–47. [ PubMed ] [ Google Scholar ]
- Karakorpi M, Alhola P, Urrila AS, et al. Hormone treatment gives no benefit against cognitive changes caused by acute sleep deprivation in postmenopausal women. Neuropsychopharmacology. 2006; 31 :2079–88. [ PubMed ] [ Google Scholar ]
- Kendall AP, Kautz MA, Russo MB, et al. Effects of sleep deprivation on lateral visual attention. Int J Neurosci. 2006; 116 :1125–38. [ PubMed ] [ Google Scholar ]
- Kilduff TS, Kushida CA, Terao A. Recovery from sleep deprivation. New York: Marcel Dekker; 2005. pp. 485–502. [ Google Scholar ]
- Killgore WD, Balkin TJ, Wesensten NJ. Impaired decision making following 49 h of sleep deprivation. J Sleep Res. 2006; 15 :7–13. [ PubMed ] [ Google Scholar ]
- Kim DJ, Lee HP, Kim MS, et al. The effect of total sleep deprivation on cognitive functions in normal adult male subjects. Int J Neurosci. 2001; 109 :127–37. [ PubMed ] [ Google Scholar ]
- Kimura D. Sex, sexual orientation and sex hormones influence human cognitive function. Curr Opin Neurobiol. 1996; 6 :259–63. [ PubMed ] [ Google Scholar ]
- Kjellberg A. Sleep deprivation and some aspects of performance. II. lapses and other attentional effects. Waking Sleeping. 1977; 1 :145–8. [ Google Scholar ]
- Kripke DF, Garfinkel L, Wingard DL, et al. Mortality associated with sleep duration and insomnia. Arch Gen Psychiatry. 2002; 59 :131–6. [ PubMed ] [ Google Scholar ]
- Kronholm E, Harma M, Hublin C, et al. Self-reported sleep duration in finnish general population. J Sleep Res. 2006; 15 :276–90. [ PubMed ] [ Google Scholar ]
- Lac G, Chamoux A. Elevated salivary cortisol levels as a result of sleep deprivation in a shift worker. Occup Med (Lond) 2003; 53 :143–5. [ PubMed ] [ Google Scholar ]
- Lee HJ, Kim L, Suh KY. Cognitive deterioration and changes of P300 during total sleep deprivation. Psychiatry Clin Neurosci. 2003; 57 :490–6. [ PubMed ] [ Google Scholar ]
- Leger D, Guilleminault C, Dreyfus JP, et al. Prevalence of insomnia in a survey of 12,778 adults in france. J Sleep Res. 2000; 9 :35–42. [ PubMed ] [ Google Scholar ]
- Linde L, Bergstrom M. The effect of one night without sleep on problem-solving and immediate recall. Psychol Res. 1992; 54 :127–36. [ PubMed ] [ Google Scholar ]
- Linde L, Edland A, Bergström M. Auditory attention and multi-attribute decision-making during a 33 h sleep-deprivation period: Mean performance and between-subject dispersions. Ergonomics. 1999; 42 :696–713. [ PubMed ] [ Google Scholar ]
- Lindheim SR, Legro RS, Bernstein L, et al. Behavioral stress responses in premenopausal and postmenopausal women and the effects of estrogen. Am J Obstet Gynecol. 1992; 167 :1831–6. [ PubMed ] [ Google Scholar ]
- Manber R, Armitage R. Sex, steroids, and sleep: A review. Sleep. 1999; 22 :540–55. [ PubMed ] [ Google Scholar ]
- Maquet P. The role of sleep in learning and memory. Science. 2001; 294 :1048–52. [ PubMed ] [ Google Scholar ]
- McCarthy ME, Waters WF. Decreased attentional responsivity during sleep deprivation: Orienting response latency, amplitude, and habituation. Sleep. 1997; 20 :115–23. [ PubMed ] [ Google Scholar ]
- Mikulincer M, Babkoff H, Caspy T, et al. The effects of 72 hours of sleep loss on psychological variables. Br J Psychol. 1989; 80 (Pt 2):145–62. [ PubMed ] [ Google Scholar ]
- Monk TH, Carrier J. Speed of mental processing in the middle of the night. Sleep. 1997; 20 :399–401. [ PubMed ] [ Google Scholar ]
- Mu Q, Nahas Z, Johnson KA, et al. Decreased cortical response to verbal working memory following sleep deprivation. Sleep. 2005; 28 :55–67. [ PubMed ] [ Google Scholar ]
- Murillo-Rodriguez E, Blanco-Centurion C, Gerashchenko D, et al. The diurnal rhythm of adenosine levels in the basal forebrain of young and old rats. Neuroscience. 2004; 123 :361–70. [ PubMed ] [ Google Scholar ]
- Naghavi HR, Nyberg L. Common fronto-parietal activity in attention, memory, and consciousness: Shared demands on integration? Conscious Cogn. 2005; 14 :390–425. [ PubMed ] [ Google Scholar ]
- National Sleep Foundation. “Sleep in America” poll. 2007. [Accessed 8 March 2007]. pp. 1–52. URL: http://www.sleepfoundation.org .
- National Sleep Foundation. “Sleep in America” poll. 2002. [Accessed 6 September 2006]. pp. 1–44. URL: http://www.sleepfoundation.org .
- Nilsson JP, Soderstrom M, Karlsson AU, et al. Less effective executive functioning after one night’s sleep deprivation. J Sleep Res. 2005; 14 :1–6. [ PubMed ] [ Google Scholar ]
- Ogawa Y, Kanbayashi T, Saito Y, et al. Total sleep deprivation elevates blood pressure through arterial baroreflex resetting: A study with microneurographic technique. Sleep. 2003; 26 :986–9. [ PubMed ] [ Google Scholar ]
- Otmani S, Pebayle T, Roge J, et al. Effect of driving duration and partial sleep deprivation on subsequent alertness and performance of car drivers. Physiol Behav. 2005; 84 :715–24. [ PubMed ] [ Google Scholar ]
- Philibert I. Sleep loss and performance in residents and nonphysicians; a meta-analytic examination. Sleep. 2005; 28 :1393–402. [ PubMed ] [ Google Scholar ]
- Philip P, Taillard J, Sagaspe P, et al. Age, performance and sleep deprivation. J Sleep Res. 2004; 13 :105–10. [ PubMed ] [ Google Scholar ]
- Pilcher JJ, Huffcutt AI. Effects of sleep deprivation on performance: A meta-analysis. Sleep. 1996; 19 :318–26. [ PubMed ] [ Google Scholar ]
- Portas CM, Rees G, Howseman AM, et al. A specific role for the thalamus in mediating the interaction of attention and arousal in humans. J Neurosci. 1998; 18 :8979–89. [ PMC free article ] [ PubMed ] [ Google Scholar ]
- Priest B, Brichard C, Aubert G, et al. Microsleep during a simplified maintenance of wakefulness test. A validation study of the OSLER test. Am J Respir Crit Care Med. 2001; 163 :1619–25. [ PubMed ] [ Google Scholar ]
- Quigley N, Green JF, Morgan D, et al. The effect of sleep deprivation on memory and psychomotor function in healthy volunteers. Hum Psychopharmacol. 2000; 15 :171–7. [ PubMed ] [ Google Scholar ]
- Ragland JD, Coleman AR, Gur RC, et al. Sex differences in brain-behavior relationships between verbal episodic memory and resting regional cerebral blood flow. Neuropsychologia. 2000; 38 :451–61. [ PMC free article ] [ PubMed ] [ Google Scholar ]
- Raidy DJ, Scharff LF. Effects of sleep deprivation on auditory and visual memory tasks. Percept Mot Skills. 2005; 101 :451–67. [ PubMed ] [ Google Scholar ]
- Rinkenauer G, Osman A, Ulrich R, et al. On the locus of speed-accuracy trade-off in reaction time: Inferences from the lateralized readiness potential. J Exp Psychol Gen. 2004; 133 :261–82. [ PubMed ] [ Google Scholar ]
- Russo MB, Kendall AP, Johnson DE, et al. Visual perception, psychomotor performance, and complex motor performance during an overnight air refueling simulated flight. Aviat Space Environ Med. 2005; 76 :C92–103. [ PubMed ] [ Google Scholar ]
- Sagaspe P, Sanchez-Ortuno M, Charles A, et al. Effects of sleep deprivation on color-word, emotional, and specific stroop interference and on self-reported anxiety. Brain Cogn. 2006; 60 :76–87. [ PubMed ] [ Google Scholar ]
- Samkoff JS, Jacques CH. A review of studies concerning effects of sleep deprivation and fatigue on residents’ performance. Acad Med. 1991; 66 :687–93. [ PubMed ] [ Google Scholar ]
- Sarter M, Gehring WJ, Kozak R. More attention must be paid: The neurobiology of attentional effort. Brain Res Brain Res Rev. 2006; 51 :145–60. [ PubMed ] [ Google Scholar ]
- Scoville WB, Milner B. Loss of recent memory after bilateral hippocampal lesions. 1957. J Neuropsychiatry Clin Neurosci. 2000; 12 :103–13. [ PubMed ] [ Google Scholar ]
- Shneerson JM. Handbook of sleep medicine. Cambridge: Blackwell Science; 2000. [ Google Scholar ]
- Smith ME, McEvoy LK, Gevins A. The impact of moderate sleep loss on neurophysiologic signals during working-memory task performance. Sleep. 2002; 25 :784–94. [ PMC free article ] [ PubMed ] [ Google Scholar ]
- Smulders FT, Kenemans JL, Jonkman LM, et al. The effects of sleep loss on task performance and the electroencephalogram in young and elderly subjects. Biol Psychol. 1997; 45 :217–39. [ PubMed ] [ Google Scholar ]
- Spiegel K, Knutson K, Leproult R, et al. Sleep loss: A novel risk factor for insulin resistance and type 2 diabetes. J Appl Physiol. 2005; 99 :2008–19. [ PubMed ] [ Google Scholar ]
- Spiegel K, Leproult R, Van Cauter E. Impact of sleep debt on metabolic and endocrine function. Lancet. 1999; 354 :1435–9. [ PubMed ] [ Google Scholar ]
- Stenuit P, Kerkhofs M. Age modulates the effects of sleep restriction in women. Sleep. 2005; 28 :1284–8. [ PubMed ] [ Google Scholar ]
- Steyvers FJ. The influence of sleep deprivation and knowledge of results on perceptual encoding. Acta Psychol (Amst) 1987; 66 :173–87. [ PubMed ] [ Google Scholar ]
- Steyvers FJ, Gaillard AW. The effects of sleep deprivation and incentives on human performance. Psychol Res. 1993; 55 :64–70. [ PubMed ] [ Google Scholar ]
- Stickgold R. Sleep-dependent memory consolidation. Nature. 2005; 437 :1272–8. [ PubMed ] [ Google Scholar ]
- Strangman G, Thompson JH, Strauss MM, et al. Functional brain imaging of a complex navigation task following one night of total sleep deprivation: A preliminary study. J Sleep Res. 2005; 14 :369–75. [ PubMed ] [ Google Scholar ]
- Swann CE, Yelland GW, Redman JR, et al. Chronic partial sleep loss increases the facilitatory role of a masked prime in a word recognition task. J Sleep Res. 2006; 15 :23–9. [ PubMed ] [ Google Scholar ]
- Taillard J, Moore N, Claustrat B, et al. Nocturnal sustained attention during sleep deprivation can be predicted by specific periods of subjective daytime alertness in normal young humans. J Sleep Res. 2006; 15 :41–5. [ PubMed ] [ Google Scholar ]
- Thomas M, Sing H, Belenky G, et al. Neural basis of alertness and cognitive performance impairments during sleepiness. I. effects of 24 h of sleep deprivation on waking human regional brain activity. J Sleep Res. 2000; 9 :335–52. [ PubMed ] [ Google Scholar ]
- Thorne DR, Thomas ML, Sing HC, et al. Driving-simulator accident rates before, during and after one week of restricted nightly sleep. Sleep. 1999; 21 :S135. [ Google Scholar ]
- Tomporowski PD, Tinsley VF. Effects of memory demand and motivation on sustained attention in young and older adults. Am J Psychol. 1996; 109 :187–204. [ PubMed ] [ Google Scholar ]
- Tsai LL, Young HY, Hsieh S, et al. Impairment of error monitoring following sleep deprivation. Sleep. 2005; 28 :707–13. [ PubMed ] [ Google Scholar ]
- Van Dongen HP, Baynard MD, Maislin G, et al. Systematic interin-dividual differences in neurobehavioral impairment from sleep loss: Evidence of trait-like differential vulnerability. Sleep. 2004; 27 :423–33. [ PubMed ] [ Google Scholar ]
- Van Dongen HP, Maislin G, Mullington JM, et al. The cumulative cost of additional wakefulness: Dose-response effects on neurobehavioral functions and sleep physiology from chronic sleep restriction and total sleep deprivation. Sleep. 2003a; 26 :117–26. [ PubMed ] [ Google Scholar ]
- Van Dongen HP, Rogers NL, Dinges DF. Sleep debt: Theoretical and empirical issues. Sleep Biol Rhythms. 2003b; 1 :5–13. [ Google Scholar ]
- Van Dongen HP, Vitellaro KM, Dinges DF. Individual differences in adult human sleep and wakefulness: Leitmotif for a research agenda. Sleep. 2005; 28 :479–96. [ PubMed ] [ Google Scholar ]
- Van Dongen HPA. Inter- and intra-individual differences in circadian phase 1998 [ Google Scholar ]
- Versace F, Cavallero C, De Min Tona G, et al. Effects of sleep reduction on spatial attention. Biol Psychol. 2006; 71 :248–55. [ PubMed ] [ Google Scholar ]
- Wilkinson RT. Response-stimulus interval in choice serial reaction time: Interaction with sleep deprivation, choice, and practice. Q J Exp Psychol A. 1990; 42 :401–23. [ PubMed ] [ Google Scholar ]
- Wimmer F, Hoffmann RF, Bonato RA, et al. The effects of sleep deprivation on divergent thinking and attention processes. J Sleep Res. 1992; 1 :223–30. [ PubMed ] [ Google Scholar ]
- Wright KP, Jr, Badia P. Effects of menstrual cycle phase and oral contraceptives on alertness, cognitive performance, and circadian rhythms during sleep deprivation. Behav Brain Res. 1999; 103 :185–94. [ PubMed ] [ Google Scholar ]
- Wu JC, Gillin JC, Buchsbaum MS, et al. The effect of sleep deprivation on cerebral glucose metabolic rate in normal humans assessed with positron emission tomography. Sleep. 1991; 14 :155–62. [ PubMed ] [ Google Scholar ]
- Zils E, Sprenger A, Heide W, et al. Differential effects of sleep deprivation on saccadic eye movements. Sleep. 2005; 28 :1109–15. [ PubMed ] [ Google Scholar ]
Sleep deprivation.
Productivity - Effect of sleep deprivation on.
Sleep deprivation
Curator: David F. Dinges
Eugene M. Izhikevich
Nick Orbeck
Tobias Denninger
Julian Lim , University of Pennsylvania, Philadelphia, PA, USA
Dr. David F. Dinges , University of Pennsylvania School of Medicine, Philadelphia, PA
Sleep deprivation is the restriction of sleep below the level of basal sleep need; this can be both acute (a single period of extended wakefulness), or chronic (the accumulation of sleep debt over multiple nights of sleep restriction). The basal sleep need of an organism is its habitual sleep duration in the absence of pre-existing sleep debt, with sleep debt defined as the duration of sleep below which waking deficits can be observed. In human beings, these deficits can be seen across a spectrum of neurocognitive domains from basic attentional processes to high-level executive function.
Under unrestricted sleep conditions, endogenous circadian and homeostatic processes interact to promote periods of stable sleep and wakefulness, with relatively abrupt transitions from one state to the other. Sleep deprivation leads to a breakdown in this stability, beginning with transient and involuntary intrusions of sleep into periods of wakefulness. These intrusions can be observed through a number of neurobehavioral phenomena, including microsleeps, sleep attacks, slow eyelid closures, voluntary naps, and slow rolling eye movements .
Apart from these directly-observable phenomena, the effects of sleep deprivation on human beings fall into two main categories: objective (which can be physiological or cognitive), and subjective.
Objective effects
Physiological.
- Sleep propensity : The classic paradigms used to measure sleep propensity are the multiple sleep latency test (MSLT) (Carskadon and Dement, 1975) and the maintenance of wakefulness test (MWT) (Mitler et al., 1982). The MSLT measures time to sleep onset when subjects are instructed to fall asleep while lying supine in a sleep-conducive environment. In contrast, the MWT measures a subject’s ability to remain awake while sitting upright in a quiet, darkened room. Sleep latency on both of these tests has an inverse relationship with the number of hours a subject has been sleep deprived.
- Endocrine changes are regularly observed in experiments of total sleep deprivation, including a reduction in levels of growth hormone (Radomski et al., 1992; Seifritz et al., 1995) and in levels of leptin in both rodents (Everson and Crowley, 2004) and humans (Mullington et al., 2003) Increases have been observed in levels of thyroid axis hormones (Allan and Czeisler, 1994; Gary et al., 1996), prolactin, luteinizing hormone and estradiol during partial sleep deprivation (Baumgartner et al., 1993). Cortisol levels, which normally follow a circadian/ultradian cycle, are altered following periods of sleep deprivation. These changes are somewhat dependent on the time of measurement post-SD; there is a rebound increase of cortisol before sleep onset after a night without sleep, (Leproult et al. 1997; Mullington et al. 1996; Weitzman et al. 1983), but decreased levels directly after the deprivation period, or when integrated over the course of the subsequent night. (Akerstedt et al. 1980; Dinges et al. 1994; Vgontzas et al. 1999a; Frey et al. 2007). Certain investigators have also found no significant change in levels of this hormone. (Gary et al. 1996; Gonzalez-Ortiz et al. 2000; Haack et al. 2002).
- Immune system/inflammatory response : Sleep deprivation has effects on the immune system, including changes in natural killer cell activity (Irwin et al., 1994; Irwin et al., 1996), interleukin-6 (Redwine et al., 2000; Shearer et al., 2001), soluble tumor necrosis factor-alpha receptor 1 (Shearer et al., 2001) and cell adhesion molecules (Frey et al., 2007). Chronic sleep deprivation in shift workers and nurses is associated with increased cardiovascular risk, possibly mediated by inflammatory markers such as C-reactive protein (Ayas et al., 2003).
- Neurogenesis : There is evidence that sleep deprivation inhibits adult neurogenesis in the dentate gyrus of the hippocampus of rodents via the action of elevated levels of circulating glucocorticoids (Hairston et al., 2005; Mirescu et al., 2006).
- Electrophysiological markers : Sleep deprivation alters the signatures of brain electrophysiology. Increasing homeostatic sleep pressure is associated with increases in spectral power in the theta band (4 -8 Hz), and decreases in the alpha band (9.25 – 10 Hz) as measured by electroencephalography (EEG). EEG changes also manifest in recovery sleep after periods of sleep deprivation, with rebound increases in slow wave activity (0.5 – 4 Hz) reported during recovery nights (Brunner et al., 1993; Van Dongen et al., 2003). It has been suggested that this EEG slow wave activity rebound, which is assumed to involve the restorative aspects of sleep, may also reflect synaptic potentiation (Tononi and Cirelli, 2003).
- Functional magnetic resonance imaging (fMRI) markers : Subjects have been tested on a variety of tasks after sleep deprivation while undergoing functional magnetic resonance imaging (fMRI). Compared to baseline, blood oxygenation level dependent (BOLD) contrast typically reveals hypoactivation in inferior parietal regions after SD (Bell-McGinty et al., 2004; Chee and Choo, 2004; Chee et al., 2006). Given that this phenomenon has been observed across a wide range of cognitive tasks, it has been suggested that parietal hypoactivation after sleep deprivation is associated with a decrease in attentional resources. However, compensatory increases in cerebral activation during sleep deprivation have also been observed during task performance (Drummond et al., 2000; Drummond et al., 2005).
- Fundamental processes : Speed of processing, as measured by neuropsychological tests such as the Digit Symbol Substitution Task, decreases with increasing time awake (Leproult et al., 1997). Additionally, tonic, sustained attention becomes increasingly labile during periods of sleep deprivation, leading to increased variability in reaction times, as well as a greater number of performance lapses on the Psychomotor Vigilance Test (PVT) and other tests of attention (Dinges et al., 1997). The time-on-task effect in sustained attention tasks is also more pronounced in the sleep-deprived state.
- Higher cognitive functions : The effects of sleep deprivation on tasks of higher cognitive function are less well known, and experimental results have been mixed. Numerous studies (Linde et al., 1999; Harrison and Horne, 2000) have shown that subjects are able to recruit compensatory resources and temporarily perform at a level comparable to that of rested controls when performing relatively more complex and engaging tasks. This is more apparent in convergent tasks (e.g. tasks of critical thinking and rule-based deduction), and less true of divergent ones (e.g. assimilation of changing information, updating strategies based on new information, lateral thinking, risk assessment, insight and temporal memory skills). Sleep loss also adversely affects working memory function, defined as the ability to encode, manipulate and retrieve information, in one or more sensory modalities (Chee and Choo, 2004). One unifying hypothesis arising from these results is that sleep deprivation differentially affects prefrontal-cortex-related functions while leaving executive ability associated with other brain regions relatively intact.
- For a more complete review of the neurocognitive consequences of sleep deprivation, refer to Durmer and Dinges (2005).
Subjective effects
- Emotion and mood : In comparison to the effects of sleep deprivation on cognition and performance, relatively little is known about the effects of sleep deprivation on emotional processes, with the exception of subjective mood states (Dinges et al., 1997). Experimental data have shown that nearly all forms of sleep deprivation result in increased negative mood states, especially feelings of fatigue, loss of vigor, sleepiness, and confusion. These data are limited however in that they were obtained without systematic mood induction procedures or other experimental probes designed to influence mood. It is not yet known if there are systematic differences in how specific events influence mood in sleepy vs. rested subjects.
Sleep deprivation is also widely believed to be associated with impaired emotional functioning. Field studies of sleep deprivation in medical personnel (Asken and Raham, 1983; Orton and Gruzeller, 1989) have found that reduced sleep is associated with increased negative and decreased positive emotional responses to specific events, but the uncontrolled nature of these studies allows for alternative interpretations of these results. Other than simple subjective self-report measures of affect, there are few laboratory-based studies on affective reactions in sleep-deprived humans.
Mathematical modeling
Interest in understanding the patterns of sleep intrusions and the other detrimental effects of sleep deprivation have led to the use and modification of existing biomathematical models of sleep-wake regulation. In particular, the two-process model of sleep regulation, first proposed by Alexander Borbély (1982), is the leading paradigm used to predict the effects of sleep and sleep deprivation on human cognition and performance. This model consists of a circadian oscillator with a period of slightly over 24 hours, as well as a homeostatic process (reflecting sleep pressure) that builds exponentially during periods of wakefulness and dissipates exponentially during periods of sleep (Figure 1). These two processes interact to promote alertness in the day and sleep during the night. It has been suggested that waking cognitive function can be mathematically modeled as the difference between the quantitative states of the circadian and homeostatic processes (Mallis, 2004).
Numerous refinements have since been proposed to improve the predictive validity of the two-process model. Adjustments have been made for environmental variables (such as light levels, and location), sleep variables (such as length and quality), and the level of cognitive workload during waking periods. In particular, including sleep inertia as an input factor improves the predictive power of the two-process model significantly. Seven leading biomathematical models of human fatigue and performance have emerged from modifications to the two-process model; these are reviewed and discussed by Mallis et al. (2004) and Dinges (2004).
Lapse and wake-state instability hypotheses
Early attempts to understand the effects of sleep deprivation focused on the “lapse hypothesis” (Dinges and Kribbs, 1991). This theory suggested that baseline levels of functioning are identical in rested and sleep-deprived states, but that sleep-deprived individuals experience transient phases of low arousal during which sleep intrusions and performance lapses occur. Although the hypothesis has some measure of explanatory power, evidence from experiments of chronic sleep restriction (Dinges et al., 1997) suggests that it is insufficient to account for the changes in neurobehavioral functioning that occur over time. For example, the increasing variability of reaction times on the Psychomotor Vigilance Test as the number of hours of sleep loss increases would not be predicted by the lapse hypothesis alone.
An alternative hypothesis is the “wake-state instability” theory, which posits that waking-state function degrades after sleep deprivation both because of lapses in attention and a decrease in tonic aspects of functioning. These global deficits in cognitive functioning account for the increased variability in performance, as well as the observed slowing of fastest or optimal responses.
Biological basis
- A pathway, once active, will tend to stay active, because it both inhibits the opposing pathway as well as the other pathway’s inhibitory effect on itself.
- A small perturbation in the system may lead to a sudden change in the pathway whose activity is dominant.
- There is strong evidence that the orexin-hypocretins are responsible for both stabilizing the sleep switch and altering its equilibrium point (from promoting wakefulness to promoting sleep and vice versa). These molecules interact directly with the arousal system, but not the VLPO, suggesting that that their action inhibits unwanted lapses into sleep (Mignot, 2004).
For a more complete review of the VLPO and its function as a sleep switch, see Saper et al. (2005).
- Adenosine has been proposed as an endogenous marker of homeostatic sleep drive. As the number of hours awake increases, brain glycogen reserves and ATP levels are depleted, with ATP degraded into ADP, AMP, and finally adenosine, which accumulates in the basal forebrain (Porkka-Heiskanen et al., 2000). Adenosine A2a receptor agonists incite VLPO neurons to express Fos protein, and also promote sleep in rats; these, and other lines of evidence (Strecker et al., 2000) point to adenosine as the driver of the negative feedback loop of sleep propensity.
- The circadian sleep-wake cycle is temporally regulated at the molecular level by the suprachiasmatic nucleus (SCN). The regulation occurs via a cyclical, 24-hour transcriptional-translational feedback loop phase set by retinal inputs that carry information about environmental light, as well as melatonin secretion from the pineal gland.
Individual differences in response
Comparisons of inter- and intra-individual variability in vulnerability to sleep deprivation have demonstrated that the former are substantially larger than the latter. Van Dongen et al. (2004) measured the intra-class coefficients (ICC) of subjective sleepiness and cognitive performance of participants undergoing three 36-hour periods of sleep deprivation. ICCs were high, with between-subject variance an order of magnitude greater than within-subject variance. These findings indicate that behavioral response to sleep deprivation is trait-like and stable over time. Leproult et al. (2003) also found that subjective and objective (EEG) measures of vulnerability to sleep deprivation were consistent when measured during separate experimental sessions.
Causes and costs
Lack of sleep, both chronic and acute, is a prevalent problem in modern society. For example, of 1.1 million Americans surveyed, as many as 20% indicated that they sleep 6.5 hours or less each night. In its extreme form, pathological levels of sleep deprivation are classified by the International Classification of Diseases (ICD) as insufficient sleep syndrome (ICD #307.49-4). Sleep deprivation can result from physiological deficits (e.g. obstructive sleep apnea ), psychological problems (e.g. secondary insomnia ), or simply reflect a lifestyle choice. Regardless of the source, it remains an under-treated symptom compared to its dramatic and often overlooked costs. For example, the cost of accidents involving sleep-deprived operators has been estimated at $43-56 billion, with almost 20% of serious motor-vehicles crashes occurring because of drowsy drivers. Sleep deprivation has also been implicated with increased risk of on-the-job errors, personal conflicts, health complaints, poorer academic performance in adolescents and increased drug use, to name just a few of its multitudinous consequences. (IOM, 2006).
- Akerstedt T, Palmblad J, de la Torre B, Marana R, Gillberg M (1980) Adrenocortical and gonadal steroids during sleep deprivation. Sleep 3:23-30.
- Allan JS, Czeisler CA (1994) Persistence of the circadian thyrotropin rhythm under constant conditions and after light-induced shifts of circadian phase. J Clin Endocrinol Metab 79:508-512.
- Asken MJ, Raham DC (1983) Resident performance and sleep deprivation: a review. J Med Educ 58:382-388.
- Ayas NT, White DP, Manson JE, Stampfer MJ, Speizer FE, Malhotra A, Hu FB (2003) A prospective study of sleep duration and coronary heart disease in women. Arch Intern Med 163:205-209.
- Baumgartner A, Dietzel M, Saletu B, Wolf R, Campos-Barros A, Graf KJ, Kurten I, Mannsmann U (1993) Influence of partial sleep deprivation on the secretion of thyrotropin, thyroid hormones, growth hormone, prolactin, luteinizing hormone, follicle stimulating hormone, and estradiol in healthy young women. Psychiatry Res 48:153-178.
- Bell-McGinty S, Habeck C, Hilton HJ, Rakitin B, Scarmeas N, Zarahn E, Flynn J, DeLaPaz R, Basner R, Stern Y (2004) Identification and differential vulnerability of a neural network in sleep deprivation. Cereb Cortex 14:496-502.
- Borbely AA (1982) A two process model of sleep regulation. Hum Neurobiol 1:195-204.
- Brunner DP, Dijk DJ, Borbely AA (1993) Repeated partial sleep deprivation progressively changes in EEG during sleep and wakefulness. Sleep 16:100-113.
- Carskadon MA, Dement WC (1975) Sleep studies on a 90-minute day. Electroencephalogr Clin Neurophysiol 39:145-155.
- Chee MW, Choo WC (2004) Functional imaging of working memory after 24 hr of total sleep deprivation. J Neurosci 24:4560-4567.
- Chee MW, Chuah LY, Venkatraman V, Chan WY, Philip P, Dinges DF (2006) Functional imaging of working memory following normal sleep and after 24 and 35 h of sleep deprivation: Correlations of fronto-parietal activation with performance. Neuroimage 31:419-428.
- Dinges DF (2004) Critical research issues in development of biomathematical models of fatigue and performance. Aviat Space Environ Med 75:A181-191.
- Dinges DF, Kribbs NB (1991) Performing while sleepy: Effects of experimentally-induced sleepiness. In: Sleep, Sleepiness and Performance (Monk TH, ed), pp 97-128. Chister, England: Wiley.
- Dinges DF, Pack F, Williams K, Gillen KA, Powell JW, Ott GE, Aptowicz C, Pack AI (1997) Cumulative sleepiness, mood disturbance, and psychomotor vigilance performance decrements during a week of sleep restricted to 4-5 hours per night. Sleep 20:267-277.
- Durmer JS, Dinges DF (2005) Neurocognitive consequences of sleep deprivation. Semin Neurol 25:117-129.
- Everson CA, Crowley WR (2004) Reductions in circulating anabolic hormones induced by sustained sleep deprivation in rats. Amer J Physiol Endocrin Metab 286(6): E1060-E1070.
- Hairston IS, Little MT, Scanlon MD, Barakat MT, Palmer TD, Sapolsky RM, Heller HC (2005) Sleep restriction suppresses neurogenesis induced by hippocampus-dependent learning. J Neurophysiol 94:4224-4233.
- Harrison Y, Horne JA (2000) The impact of sleep deprivation on decision making: a review. J Exp Psychol Appl 6:236-249.
- IOM (2006) Sleep Disorders and Sleep Deprivation: An Unmet Public Health Problem. Washington DC: National Academies Press.
- Irwin M, Mascovich A, Gillin JC, Willoughby R, Pike J, Smith TL (1994) Partial sleep deprivation reduces natural killer cell activity in humans. Psychosom Med 56:493-498.
- Irwin M, McClintick J, Costlow C, Fortner M, White J, Gillin JC (1996) Partial night sleep deprivation reduces natural killer and cellular immune responses in humans. Faseb J 10:643-653.
- Leproult R, Van Reeth O, Byrne MM, Sturis J, Van Cauter E (1997) Sleepiness, performance, and neuroendocrine function during sleep deprivation: effects of exposure to bright light or exercise. J Biol Rhythms 12:245-258.
- Leproult R, Colecchia EF, Berardi AM, Stickgold R, Kosslyn SM, Van Cauter E (2003) Individual differences in subjective and objective alertness during sleep deprivation are stable and unrelated. Am J Physiol Regul Integr Comp Physiol 284:R280-290.
- Linde L, Edland A, Bergstrom M (1999) Auditory attention and multiattribute decision-making during a 33 h sleep-deprivation period: mean performance and between-subject dispersions. Ergonomics 42:696-713.
- Mallis MM, Mejdal S, Nguyen TT, Dinges DF (2004) Summary of the key features of seven biomathematical models of human fatigue and performance. Aviat Space Environ Med 75:A4-14.
- Mignot E (2004) Sleep, sleep disorders and hypocretin (orexin). Sleep Med 5 Suppl 1:S2-8.
- Mirescu C, Peters JD, Noiman L, Gould E (2006) Sleep deprivation inhibits adult neurogenesis in the hippocampus by elevating glucocorticoids. Proc Natl Acad Sci U S A 103:19170-19175.
- Mitler MM, Gujavarty KS, Browman CP (1982) Maintenance of wakefulness test: a polysomnographic technique for evaluation treatment efficacy in patients with excessive somnolence. Electroencephalogr Clin Neurophysiol 53:658-661.
- Mullington JM, Chan JL, Van Dongen HP, Szuba MP, Samaras J, Price NJ, Meier-Ewert HK, Dinges DF, Mantzoros CS (2003) Sleep loss reduces diurnal rhythm amplitude of leptin in healthy men. J Neuroendocrinol 15:851-854.
- Orton DI, Gruzeller JH (1989) Adverse changes in mood and cognitive performance of house officers after night duty. Br Med J 298:21-23.
- Porkka-Heiskanen T, Strecker RE, McCarley RW (2000) Brain site-specificity of extracellular adenosine concentration changes during sleep deprivation and spontaneous sleep: an in vivo microdialysis study. Neuroscience 99:507-517.
- Radomski MW, Hart LE, Goodman JM, Plyley MJ (1992) Aerobic fitness and hormonal responses to prolonged sleep deprivation and sustained mental work. Aviat Space Environ Med 63:101-106.
- Redwine L, Hauger RL, Gillin JC, Irwin M (2000) Effects of sleep and sleep deprivation on interleukin-6, growth hormone, cortisol, and melatonin levels in humans. J Clin Endocrinol Metab 85:3597-3603.
- Saper CB, Scammell TE, Lu J (2005) Hypothalamic regulation of sleep and circadian rhythms. Nature 437:1257-1263.
- Seifritz E, Hemmeter U, Trachsel L, Lauer CJ, Hatzinger M, Emrich HM, Holsboer F, Holsboer-Trachsler E (1995) Effects of flumazenil on recovery sleep and hormonal secretion after sleep deprivation in male controls. Psychopharmacology (Berl) 120:449-456.
- Shearer WT, Reuben JM, Mullington JM, Price NJ, Lee BN, Smith EO, Szuba MP, Van Dongen HP, Dinges DF (2001) Soluble TNF-alpha receptor 1 and IL-6 plasma levels in humans subjected to the sleep deprivation model of spaceflight. J Allergy Clin Immunol 107:165-170.
- Spiegel K, Leproult R, Van Cauter E (1999) Impact of sleep debt on metabolic and endocrine function. Lancet 354:1435-1439.
- Strecker RE, Morairty S, Thakkar MM, Porkka-Heiskanen T, Basheer R, Dauphin LJ, Rainnie DG, Portas CM, Greene RW, McCarley RW (2000) Adenosinergic modulation of basal forebrain and preoptic/anterior hypothalamic neuronal activity in the control of behavioral state. Behav Brain Res 115:183-204.
- Tononi G, Cirelli C (2003) Sleep and synaptic homeostasis: a hypothesis. Brain Res Bull 62:143-150.
- Van Dongen HP, Maislin G, Mullington JM, Dinges DF (2003) The cumulative cost of additional wakefulness: dose-response effects on neurobehavioral functions and sleep physiology from chronic sleep restriction and total sleep deprivation. Sleep 26:117-126.
- Van Dongen HP, Baynard MD, Maislin G, Dinges DF (2004) Systematic interindividual differences in neurobehavioral impairment from sleep loss: evidence of trait-like differential vulnerability. Sleep 27:423-433.
- Weitzman ED, Zimmerman JC, Czeisler CA, Ronda J (1983) Cortisol secretion is inhibited during sleep in normal man. J Clin Endocrinol Metab 56:352-358.
Internal references
- James B. Aimone, Sebastian Jessberger, Fred H. Gage (2007) Adult neurogenesis . Scholarpedia , 2(2):2100.
- Valentino Braitenberg (2007) Brain . Scholarpedia, 2(11):2918.
- Paul L. Nunez and Ramesh Srinivasan (2007) Electroencephalogram . Scholarpedia, 2(2):1348.
- Eugene M. Izhikevich (2007) Equilibrium . Scholarpedia, 2(10):2014.
- Keith Rayner and Monica Castelhano (2007) Eye movements . Scholarpedia, 2(10):3649.
- Seiji Ogawa and Yul-Wan Sung (2007) Functional magnetic resonance imaging . Scholarpedia, 2(10):3105.
- Jeff Moehlis, Kresimir Josic, Eric T. Shea-Brown (2006) Periodic orbit . Scholarpedia, 1(7):1358.
- John Dowling (2007) Retina . Scholarpedia, 2(12):3487.
- Philip Holmes and Eric T. Shea-Brown (2006) Stability . Scholarpedia, 1(10):1838.
- S. Murray Sherman (2006) Thalamus . Scholarpedia, 1(9):1583.
- Olivier Walusinski (2008) Yawn , Scholarpedia, 3(6):6463.
External links
- David F. Dinges's website
Insomnia , Sleep , Sleep Apnea
- Neuroscience
- Multiple Curators
Personal tools
- View source
- View history
- Propose a new article
- Instructions for Authors
- Random article
Focal areas
- Astrophysics
- Celestial mechanics
- Computational neuroscience
- Computational intelligence
- Dynamical systems
- More topics
- Recently published articles
- Recently sponsored articles
- Recent changes
- All articles
- List all Curators
- List all users
- Scholarpedia Journal
- What links here
- Related changes
- Special pages
- Printable version
- Permanent link

- "Sleep deprivation" by Julian Lim and David F. Dinges is licensed under a Creative Commons Attribution-NonCommercial-ShareAlike 3.0 Unported License . Permissions beyond the scope of this license are described in the Terms of Use
- Privacy policy
- About Scholarpedia
- Disclaimers
- Type 2 Diabetes
- Heart Disease
- Digestive Health
- Multiple Sclerosis
- COVID-19 Vaccines
- Occupational Therapy
- Healthy Aging
- Health Insurance
- Public Health
- Patient Rights
- Caregivers & Loved Ones
- End of Life Concerns
- Health News
- Thyroid Test Analyzer
- Doctor Discussion Guides
- Hemoglobin A1c Test Analyzer
- Lipid Test Analyzer
- Complete Blood Count (CBC) Analyzer
- What to Buy
- Editorial Process
- Meet Our Medical Expert Board
Restorative Theory and More Ideas About Why We Sleep
Verywell / Hetal Rathod
Restorative Theory
Adaptive theory.
- Energy Conservation
- Brain Plasticity
Frequently Asked Questions
Even though it's something you have to do every day, why we sleep remains a mystery. Many sleep theories abound, yet scientists are far from universal agreement about how to answer the question, "Why do we sleep at night?" Only in the last few decades have they even begun to unravel sleep's true secrets. At least four common theories are in play, but it remains to be seen which—if any—are correct.
You may look at these theories and the scientific evidence that supports them and wonder why they all can't, at least in part, be right. They could be, but researchers are continuing to search for "the" core reason for sleep, and many subscribe to a belief expressed in a 1998 paper:
Allen Rechtschaffen
Sleep can be understood as fulfilling many different functions but intuition suggests there is one essential function. The discovery of this function will open an important door to the understanding of biological processes.
The sleep cycle involves several stages, but these theories generally focus on rapid-eye movement (REM) sleep —which is when you dream —and the other stages lumped together as non-REM sleep.
The restorative theory of sleep, first proposed in 2006, is among the more accepted explanations for why people need sleep. It suggests that the purpose of sleep is to store memory and restore our brains and bodies for the next day.
- Organizing and storing memories : Memories are believed to be converted from short-term to long-term storage, while information deemed unimportant is removed. This primarily occurs during REM sleep, which is when the brain cells most involved in memory, attention, and learning are least active.
- Clearing out and replenishing brain chemicals : Many brain chemicals build up while you're awake, including adenosine , which makes you sleepy when it accumulates. Meanwhile, during sleep, the brain "restocks" the chemicals it uses for sending signals and other purposes, so you have enough for the next day.
- Clearing waste toxins from the brain : Similar to brain chemicals, the waste products of energy metabolism build up during the day and are cleared out at night. (However, the primary evidence for this comes from studies of mice, not humans.)
- Repairing tissues throughout the body : During sleep, your body increases cellular division, growth hormone levels, and the production of proteins necessary for proper function. This is believed to be why strenuous physical activity during the day increases your time in REM sleep.
- Rest for the brain : The inactivity during non-REM sleep appears to provide a period of rest for the brain.
Some of the brain chemicals that accumulate during the day are associated with the plaques that characterize Alzheimer's disease , so it's theorized that the brain-clearing activities of sleep may protect you against Alzheimer's.
Also called the evolution theory or preservation theory, the original version of this early theory suggested that while humans evolved, hiding out overnight increased the ability to survive.
For our early ancestors, nighttime was dangerous—especially because the predators who hunt at night function better in the dark than humans do—so it made sense to seek a safe refuge. Also, because they couldn't be out finding food themselves, their bodies slowed down to conserve energy for when they could be active. People who avoided dangers in this way, the theory argued, lived longer and were more likely to reproduce.
Thus, sleep became an adaptive or evolutionary advantage and became part of the neurochemistry of the species. However, most experts reject this idea because sleeping leaves animals (including humans) vulnerable and defenseless, which doesn't support the idea that sleeping made our ancestors safer.
A flaw in this theory, some experts say, is that sleeping limits productivity, such as finding food and reproducing, so staying awake longer would be an evolutionary benefit. However, no species evolved without a need for sleep, which calls the adaptive benefit into question.
According to some sleep researchers, the daily need for sleep combined with the incentive not to be out in the dark caused us to adapt to function best during daylight, which prevented us from adapting to the dark.
Energy Conservation Theory
In a similar vein to aspects of other theories, some experts theorize the primary purpose of sleep is conserving energy. By sleeping, they say, you're able to spend part of your time functioning at a lower metabolism.
That lowers the number of calories you need to eat. For early humans, that extra food requirement could have been the difference between life and death, or survival of the species versus extinction. It was harder to gather food at night, so it made sense to stay hidden then. They also point to the brain's need to replenish its reserve of glycogen, which is an important fuel.
However, while it's true that metabolism slows during non-REM, the brain is extremely active during REM sleep, which some say is a strike against the energy conservation theory.
Brain Plasticity Theory
Among the more recent theories deals with brain plasticity (also called neuroplasticity), which is the brain's ability to change and adapt in response to experience. It can change both functional aspects (such as re-learning skills in a new area after damage) and structural aspects (such as forming new pathways due to learning).
The brain plasticity theory says that sleep is necessary for the brain to make structural changes. Support for this theory comes from many places.
As in restorative theory, this concept deals with information processing and memory formation. Research suggests that sleep loss leads to less structural plasticity, which can have a negative effect on alertness, cognition, and mood. Sleep deprivation also compromises memory formation, which is related to learning and plasticity.
It's believed that the plasticity theory explains why babies and young children require a lot of sleep—they're learning so much about the world that their brains need more time to process it. Researchers are even trying to promote less sleep interruption for preterm babies in neonatal intensive care, citing studies about sleep's long-term impact on brain development and plasticity.
Some researchers have even theorized that sleep is the price we pay for brain plasticity. That concept is based on the importance of the processes that occur during sleep to the brain's ability to adapt and change.
Declining Sleep Needs
Newborns need between 14 and 17 hours of sleep per day. The recommended amount of sleep declines throughout childhood, with teenagers needing between 8 and 10 hours a day.
Why do we close our eyes when we sleep?
There are several reasons why most people sleep with eyes closed, though some people do actually sleep with their eyes open. Closed eyes stay moist and are protected by the eyelids during sleep. Eyelids also block out light during sleep, and light signals to the brain that it is time to wake up.
At what stage of sleep can you experience sleep paralysis?
Sleep paralysis , or the feeling that you are conscious and yet unable to control your body, is very common and typically occurs during the transition from REM to being awake.
Why do we dream?
There are a lot of theories explaining why we dream, such as that dreaming aids in memory processing and that it allows us to process emotions. Sigmund Freud believed that dreams express our unconscious desires and deepest wishes.
A Word From Verywell
While it's a phenomenon we don't fully understand, sleep is critical to our daily health. Not only is it necessary for restoration and repair, learning and memory, growth and development, and brain plasticity, sleep also helps with problem-solving, a healthy metabolism, blood-sugar and hormone regulation, heart health, and strengthening immunity. With how crucial it is to our survival, it's no wonder many of us long for more of it.
Freiberg AS. Why we sleep: A hypothesis for an ultimate or evolutionary origin for sleep and other physiological rhythms . J Circadian Rhythms . 2020;18:2. Published 2020 Mar 30. doi:10.5334/jcr.189
Ezenwanne E. Current concepts in the neurophysiologic basis of sleep; a review . Ann Med Health Sci Res ; 1(2):173-179.
Xie L, Kang H, Xu Q, et al. Sleep drives metabolite clearance from the adult brain . Science . 2013;342(6156):373–377. doi:10.1126/science.1241224
Raven F, Van der Zee EA, Meerlo P, Havekes R. The role of sleep in regulating structural plasticity and synaptic strength: Implications for memory and cognitive function . Sleep Med Rev . 2018;39:3-11. doi:10.1016/j.smrv.2017.05.002
Park J. Sleep promotion for preterm infants in the NICU . Nurs Womens Health . 2020;24(1):24-35. doi:10.1016/j.nwh.2019.11.004
Tononi G, Cirelli C. Sleep and the price of plasticity: From synaptic and cellular homeostasis to memory consolidation and integration . Neuron . 2014;81(1):12-34. doi:10.1016/j.neuron.2013.12.025
U.S. Centers for Disease Control and Prevention. Sleep and sleep disorders: How much sleep do I need?
National Sleep Foundation. Can you really sleep with your eyes open ?
Rasch B, Born J. About sleep's role in memory . Physiol Rev . 2013;93(2):681-766. doi:10.1152/physrev.00032.2012
Zhang W, Guo B. Freud's dream interpretation: A different perspective based on the self-organization theory of dreaming . Front Psychol . 2018;9:1553. doi:10.3389/fpsyg.2018.01553
National Institutes of Health, National Heart, Lung, and Blood Institute. Sleep deprivation and deficiency .
By Brandon Peters, MD Brandon Peters, MD, is a board-certified neurologist and sleep medicine specialist.
Thank you for visiting nature.com. You are using a browser version with limited support for CSS. To obtain the best experience, we recommend you use a more up to date browser (or turn off compatibility mode in Internet Explorer). In the meantime, to ensure continued support, we are displaying the site without styles and JavaScript.
- View all journals
- Explore content
- About the journal
- Publish with us
- Sign up for alerts
- Neuropsychopharmacology Reviews
- Published: 09 May 2019
Sleep, insomnia, and depression
- Dieter Riemann ORCID: orcid.org/0000-0002-1968-6220 1 ,
- Lukas B. Krone 2 , 3 ,
- Katharina Wulff 4 , 5 &
- Christoph Nissen ORCID: orcid.org/0000-0001-9809-0275 6
Neuropsychopharmacology volume 45 , pages 74–89 ( 2020 ) Cite this article
42k Accesses
337 Citations
83 Altmetric
Metrics details
- Circadian rhythms and sleep
- Predictive markers
Since ancient times it is known that melancholia and sleep disturbances co-occur. The introduction of polysomnography into psychiatric research confirmed a disturbance of sleep continuity in patients with depression, revealing not only a decrease in Slow Wave Sleep, but also a disinhibition of REM (rapid eye movement) sleep, demonstrated as a shortening of REM latency, an increase of REM density, as well as total REM sleep time. Initial hopes that these abnormalities of REM sleep may serve as differential-diagnostic markers for subtypes of depression were not fulfilled. Almost all antidepressant agents suppress REM sleep and a time-and-dose–response relationship between total REM sleep suppression and therapeutic response to treatment seemed apparent. The so-called Cholinergic REM Induction Test revealed that REM sleep abnormalities can be mimicked by administration of cholinomimetic agents. Another important research avenue is the study of chrono-medical timing of sleep deprivation and light exposure for their positive effects on mood in depression. Present day research takes the view on insomnia, i.e., prolonged sleep latency, problems to maintain sleep, and early morning awakening, as a transdiagnostic symptom for many mental disorders, being most closely related to depression. Studying insomnia from different angles as a transdiagnostic phenotype has opened many new perspectives for research into mechanisms but also for clinical practice. Thus, the question is: can the early and adequate treatment of insomnia prevent depression? This article will link current understanding about sleep regulatory mechanisms with knowledge about changes in physiology due to depression. The review aims to draw the attention to current and future strategies in research and clinical practice to the benefits of sleep and depression therapeutics.
You have full access to this article via your institution.
Similar content being viewed by others
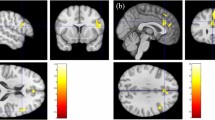
The moderating effect of prefrontal response to sleep-related stimuli on the association between depression and sleep disturbance in insomnia disorder
Mi Hyun Lee, Kyung Hwa Lee, … Yu Jin Lee
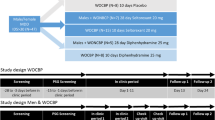
The selective orexin-2 antagonist seltorexant (JNJ-42847922/MIN-202) shows antidepressant and sleep-promoting effects in patients with major depressive disorder
Kasper Recourt, Peter de Boer, … Wayne Drevets
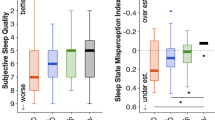
Insomnia subtypes characterised by objective sleep duration and NREM spectral power and the effect of acute sleep restriction: an exploratory analysis
Chien-Hui Kao, Angela L. D’Rozario, … Christopher J. Gordon
Introduction—the phenomenology of sleep in depression
A brief historical overview.
Robert Burton, in his Anatomy of Melancholia [ 1 ] remarked that ancient Greek physicians were well aware of the fact that melancholic individuals complained of difficulties falling asleep, maintaining sleep or of waking up too early in the morning. Treatment of difficulties with sleep in antique times consisted of listening to calm music, reading, or the use of opium or alcohol [ 2 ]. Emil Kraepelin, the founder of modern psychiatry, observed that mental symptoms fall mainly into two groups and he created the illness categories [ 3 ] ‘manic-depression’’ and ‘dementia praecox’’, today clinically similar to ‘affective’’ and ‘psychotic’’ phenotypes. He postulated that a certain type of sleep difficulty co-occurred with a certain depressive subtype, i.e., sleep onset problems with “neurotic” and sleep maintenance/early morning awakenings with “endogenous” depression. Thus, the idea that the type of sleep disturbance in depressed individuals might support differential-diagnostic considerations was already born over a 100 years ago and experienced a renaissance in the 1970s with the discovery of shortened rapid eye movement (REM) sleep latencies in depressed individuals. Before then, pharmacological agents like bromides, paraldehyde, scopolamine, or barbiturates were used to treat insomnia accompanying depression until these were replaced by benzodiazepine hypnotics and sedating antidepressants in the 1950/60s. The incidence of depression is steadily rising globally and present-day epidemiological data reveal that depression is now the third leading contribution to global disease burden [ 4 ] and an estimated 25% of the population in industrialized countries will suffer from depression once in a lifetime [ 5 ]. This review seamlessly ties in with and extends an earlier line of research of using sleep as a window into the brain’s neurobiological links between sleep processes and depression. By telling the story of an early success, a subsequent stagnation phase and new enthusiasm based on the integration of emerging neurobiological knowledge into the mechanistic overlap of sleep and depression disturbances, this review is paving a way for the integration of this knowledge into clinical practice.
REM sleep and depression—early hopes
The discovery of phases of REM during sleep in 1953 [ 6 ] and the ensuing interest in sleep research led to the establishment of psychiatric sleep research utilizing polysomnography (PSG). Kupfer et al. from Pittsburgh [ 7 , 8 ] were among the first to suggest that changes of REM sleep, i.e., shortened REM sleep latency (shorter interval between sleep onset and the first occurrence of REM sleep), increased total REM sleep duration and increased REM density (higher phasic eyes movements during REM sleep), are typical sleep characteristics of patients with primary vs. secondary depression (see Fig. 1 for illustration). Furthermore, polysomnographically measured sleep continuity was disturbed (i.e., long sleep latency and frequent awakenings throughout the sleep period) and Slow Wave Sleep (SWS) reduced. These findings were met with enthusiasm at the time and promoted the idea, later known as biomarkers [ 9 ], to identify functional subtypes within and across diagnostic categories by which treatment could be stratified and response predicted for each patient in order to achieve remission.
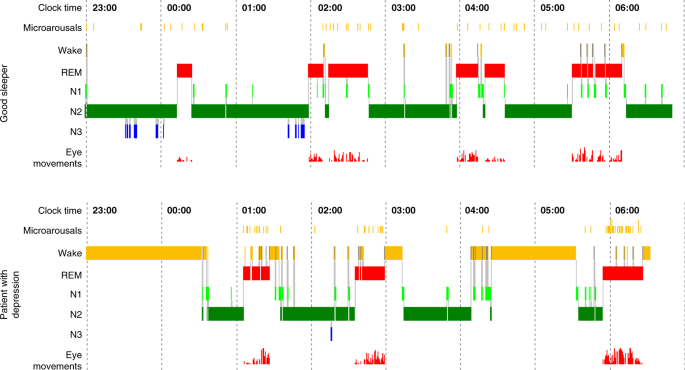
Comparison of the polysomnographic (PSG) profile of a good sleeper (upper panel) and a patient hospitalized for severe depression according to DSM-IV criteria (lower panel). Both subjects have been free from intake of any psychotropic drug for at least 14 days. The y -axis lists arousal (micro-arousals), wake and sleep stages (REM, stage N1 to N3) and eye movements). The x -axis is the time axis. Sleep in depression is characterized by alterations of sleep continuity (prolonged sleep onset and sleep maintenance problems), a decrement of SWS (Slow Wave Sleep, also measurable as a decline in Delta-Power) and a disinhibition of REM sleep: this encompasses shortening of REM latency, prolongation of the first REM period and increase of REM density. Original data from Freiburg sleep lab, hitherto unpublished
REM sleep and depression—disappointed expectations
The question of the differential-diagnostic value of REM sleep abnormalities puzzled psychiatric sleep research for almost two decades [ 10 ]. It was thought that REM sleep abnormalities might be more typical for the ‘primary/melancholic/endogenous’’ subtype compared to the ‘secondary/neurotic/non-melancholic’’ subtype. With increasing research efforts, however, it turned out that especially age, and to a certain extent sex, has a very strong effect on REM sleep latency, whereby REM sleep latency decreases with age. Revisiting the primary/secondary dichotomy and REM latency, carefully controlling for age and severity of depression, Thase et al. [ 11 ] concluded that initial positive findings may have represented epiphenomena of sample differences with respect to age or severity of depression. Additional research into other mental disorders (especially schizophrenia, borderline personality disorder and alcohol dependency) revealed that patients with these diagnostic entities also display some degree of REM sleep alterations [ 12 , 13 ], further weakening the assumptions of a high specificity of REM sleep abnormalities for depression.
Antidepressants and sleep in depression
An important impetus for the field of sleep research in depression was the observation that almost all antidepressants influence sleep, notably by strongly suppressing REM sleep [ 14 ], whereby the extent of REM sleep suppression covaried with clinical ratings indicating therapeutic efficacy of antidepressants response [ 10 ]. Thus, REM suppression appeared as a promising early predictor of subsequent treatment response. Identifying such early predictor that could function as a biomarker would be of high clinical value given the long latency to response and the limited response rate of ~60% of depressed patients. Insofar, the suppression of REM sleep seemed to constitute a window to the brain reflecting therapeutic efficacy of antidepressant substances. Table 1 summarizes the effects of antidepressants on different aspects of polysomnographically recorded sleep [ 14 ].
Data in the table demonstrate that most antidepressants suppress REM sleep, with only a few exceptions such as trimipramine, trazodone and mirtazapine. Many of these substances lead to an enhanced SWS and improved sleep continuity but it is important to note that certain drug classes, especially SSRIs (Selective Serotonin Reuptake Inhibitors), may induce a deterioration of sleep continuity, which is clinically expressed as increased insomnia complaints. This heterogeneity posed a challenge to the initial hope of using PSG to predict treatment response and it has been given up by and large [ 14 ]—partly due to failures to replicate the predictive value of REM sleep suppression for later therapeutic response, partly due to the inherent costs and clinical impracticability of using PSG as a drug monitoring tool. Today, miniaturized EEG monitors and refined semi-automatic algorithms for sleep analyses might allow the introduction of sleep EEG into the clinics [ 15 ]. An intrinsic value of this line of research, however, is that it is now widely acknowledged that drug wash-out periods of at least 7–14 days (for fluoxetine even longer) are needed to obtain valid sleep data. This, however, renders PSG research into depression complicated, because today most patients with severe depression who come into contact with a research facility are pre-medicated and drug-withdrawal would not be consistent with standard guidelines and considered unethical.
The cholinergic REM induction test
An experimental avenue towards a mechanistic understanding of REM sleep abnormalities in depression constituted the Cholinergic REM Induction Test (CRIT). This strategy, developed by Gillin and Sitaram [ 16 ], was based on the reciprocal interaction model of Non-REM/REM sleep regulation [ 17 , 18 ] positing that REM sleep onset can be advanced in animals and healthy volunteers by cholinergic stimulation [ 19 ] because REM sleep is largely governed by cholinergic neurons in the brain stem (see Fig. 2 ).
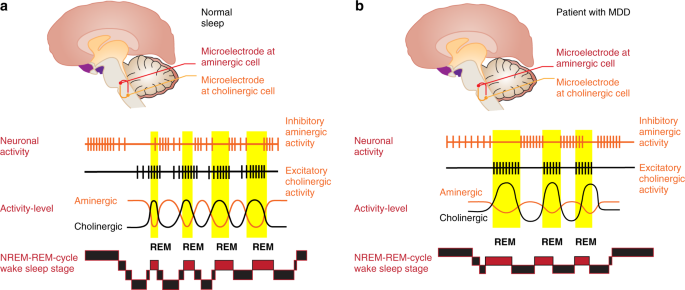
a , b The Hobson/McCarley Model and the regulation of Non-REM and REM sleep in good sleepers and patients with depression. Experiments by Hobson and McCarley in cats especially in the brain stem were able to show that manipulation of cholinergic or aminergic cell groups is able to change sleep. In the case of good sleepers ( a ) the aminergic systems (dorsal raphe/locus coeruleus) are active during Non-REM sleep and cease their activity around the onset of REM sleep, where cholinergic activity becomes dominant. The reciprocal interaction between the aminergic and the cholinergic system determines the Non-REM-REM cycle. In depression ( b ), an overactive cholinergic system or a weakened aminergic system leads to an earlier onset of REM sleep. Based on refs [ 10 , 18 ], permission granted
Berger et al. [ 20 ] showed that such a cholinergic stimulus elicited an even more-pronounced response in patients with depression compared to good sleepers and patients with other mental disorders. Other studies showed that this early onset of REM sleep may also be provoked by cholinomimetic agents in individuals at high risk for depression [ 21 ], who later displayed a higher rate of episodes of depression over a follow-up period of >10 years [ 22 ]. Thus, it was demonstrated that early onset of REM sleep after cholinergic stimulation is not only a state and trait marker, but also a vulnerability marker for depression. Given that drug wash-out periods of at least 7–14 days are necessary to obtain valid CRIT data, it is understandable that, apart from its scientific value, the CRIT never saw widespread clinical application for determining risk profiles.
Biological timing and sleep deprivation in depression
Wehr et al. [ 23 ] published ground-breaking work on the likely involvement of the biological time keeping system in the pathogenesis of affective disorders, high-lighting that an advance of the sleep period by 6 h normalized the REM sleep phases and induced a longer-lasting remission of depressive symptoms. The authors inferred from their longitudinal observations that REM sleep rhythmicity and their underlying biochemical rhythms must have altered internal phase-relationships that are associated with certain psychopathological phenotypes. Similar remissions of depressive symptoms were also achieved in patients undergoing complete wake therapy (full night of sleep deprivation), that was first tested by Pflug and Toelle [ 24 ]. These results were better understood with the two-process model of sleep regulation proposed by Borbély et al. [ 25 , 26 , 27 ], in which a homeostatic sleep process (S) and a circadian process (C), interact in a threshold- and time-dependent manner (see Fig. 3 ), thereby incorporating that appropriate timing of sleep with respect to the internal clock is crucial for stable mood as described in the ‘internal coincidence’’ model [ 28 , 29 ].
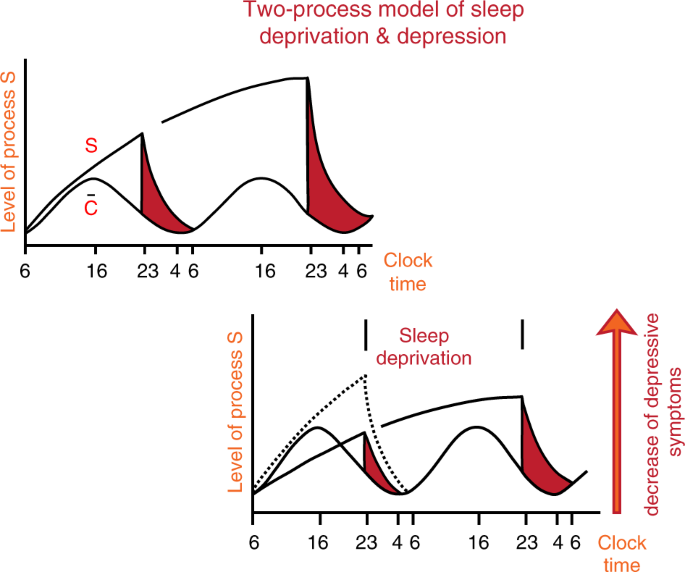
The Two-Process-Model is based on two components or factors, i.e., process C and process S. Process C reflects circadian rhythmicity, which is under control of the light–dark cycle. Process S reflects sleep or sleep pressure and can be measured as delta waves during night sleep. The interaction between C and S describes or determines the sleep-wake cycle in good sleepers (upper left panel). In contrast, in depression (lower right panel), process S is deficient, as reflected by low levels of slow wave sleep/delta power in the spectral analysis of the sleep EEG. Sleep deprivation may act antidepressive because it enhances process S, thus leading to an increased amount of slow wave sleep after sleep deprivation. Personal permission by A. Borbely
Germain and Kupfer [ 30 ] summarized further chronobiologically inspired experiments based on circadian/ sleep hypotheses of depression, including the circadian phase-shift effects of light [ 31 ] or the social rhythm model [ 32 ]. At present, the most comprehensive, decisive review on sleep deprivation therapy explaining its antidepressant effects on the level of molecular circadian regulation was compiled by Bunney and Bunney [ 33 ]. Clinically, therapeutic sleep deprivation (SD) is a rapid acting treatment for a subset of patients with major depression. Within 24 h it provokes a transient, but strong decrease in depressive symptoms in those who respond [ 34 , 35 ]. Unfortunately, the effect is not long lasting and a relapse into depression occurs after the next night of sleep. Even brief daytime sleep periods after SD can reverse the therapeutic effect [ 36 ]. Strategies to enhance or prolong the antidepressive effect are concomitant pharmacotherapy, light therapy or sleep phase advance, if correctly timed and any deviation from the schedule avoided to prevent a rebound into depressive symptomatology. Paradigms of SD in depression include total sleep deprivation, partial sleep deprivation (mostly concerning the second half of the night), selective REM sleep deprivation and selective slow wave sleep (SWS) deprivation [ 37 ]. Clinical predictors for positive SD response include the melancholic subtype of depression, a high level of vigilance, the propensity to produce diurnal mood variations and short REM latency [ 38 , 39 ]. From a scientific point of view, SD presents a unique paradigm to study the neurobiology of major depression within a short period of time and without pharmacological interference because patients can be investigated in a depressed and non-depressed/euthymic state. Unfortunately, up to now, the neurobiological basis of the immediate antidepressant response to SD and its rapid drop with more sleep is not sufficiently understood. The rapid antidepressive mechanisms probably is quite different from conventional treatments (pharmacological/psychotherapy). Therefore, elucidating the neuronal mechanisms of SD has become crucially important for novel perspectives on antidepressive treatment.
We recently integrated the synaptic plasticity hypothesis of major depression [ 40 , 41 ] and the synaptic homeostasis hypothesis of sleep-wake regulation [ 42 , 43 ] into a synaptic plasticity model of sleep wake regulation. The model posits, in brief, that sleep deprivation, through an increase of overall synaptic strength, shifts patients with major depression into a more favorable window of synaptic plasticity and network function [ 44 ]. While certainly oversimplified, the model allows for testing specific predictions, such as proposed antidepressive properties of selective slow wave sleep suppression, for instance through auditory closed-loop stimulation [ 45 , 46 ] and translation into animal models of depression for further deciphering the neural mechanisms of rapid treatment response.
Sleep and depression: neuroimaging results
Advances in human brain imaging techniques, particularly [18F]2-fluoro-2-deoxy-d-glucose PET (Positron Emission Tomography) studies, have led to new insights into changes in brain metabolism during the sleep-wake cycle in healthy humans and those with depression, which are not accessible to surface electrophysiology. The integration of these studies suggests that functional neuroanatomic correlates can be assigned to characteristic PSG alterations in depression, of which three main components can be distinguished. First, persistent hyperactivity in the basic ascending arousal system throughout the sleep-wake cycle in depression might be implicated in the experience of hyperarousal during waking and disturbed sleep continuity [ 47 ]. Second, persistent hyperactivity in the ventral emotional system that includes the amygdala and the ventral anterior cingulate cortex (ACC) might relate to disturbances in affect, including depressed mood, and to the characteristic enhancement of REM sleep in depression [ 48 ]. Third, hypoactivity in the dorsal executive system throughout the sleep-wake cycle that includes the dorsolateral prefrontal cortex (DLPFC) might be implicated in the attenuation of executive functions and reduced slow-wave sleep in depression [ 49 ]. It is of note that these observations do not directly inform about the direction of causality. Interventional studies, such as non-invasive brain stimulation studies, would be needed to test whether the described alterations of sleep in depression can be corrected and whether this kind of non-invasive stimulation might exert therapeutic effects.
Interim summary
Sleep and circadian rhythm research in psychiatry had temporarily lost much of its momentum over the disappointment of the unspecificity of REM sleep alterations for depression [ 12 , 13 ], as well as due to the introduction of more easy-to-apply neuroimaging methods in psychiatry, and the rise of SSRIs as mainstay treatment for affective disorders. Promising strategies such as the cholinergic REM induction test or the application of PET to study sleep in depression provided highly interesting and exciting results, but were not followed-up because of their immense economic and time-consuming costs that precluded the introduction of these techniques into clinical routine. The fact that practically all available antidepressive medications have more or less strong effects on the physiology of sleep created another roadblock. Today, researchers are confronted with mainly pre-medicated patients for whom drug discontinuation (with a wash-out periods of at least 7–14 days) for research purposes would be considered unethical. A further big hurdle for sleep research (but indeed probably for all types of biological psychiatric research) constituted the fact that a categorical approach to nosology prevailed, hampering any biologically meaningful investigation into psychophysiological underlying mechanisms across diagnostic boundaries. This obstacle has finally been overcome by overwhelming evidence of transdiagnostic and dimensional constellations in pathophysiological traits. The transdiagnostic role of insomnia for many mental disorders, and especially considering its strong association with depression, either as a symptom, syndrome or a distinct diagnostic entity in itself, has become a viable strategy to investigate the sleep—depression relationship. It is our hope that this paradigm change in research may also be clinically more fruitful with respect to aspects of detection, diagnosis and treatment of depression.
The relationship between insomnia and depression
The previous chapters on the phenomenology of sleep and depression have exemplified that disturbances of sleep continuity ubiquitously accompany affective disorders. Whereas the low specificity of insomnia symptoms rendered the study of insomnia unattractive for years, today symptoms of insomnia are seen as a critical feature of depression and a better understanding of the processes involved should contribute to the refinement of pathophysiological concepts and therapeutic approaches for depressive disorders.
What is insomnia?
DSM-5 has given up the distinction between primary and secondary insomnia and now includes the diagnostic category “insomnia disorder” [ 50 ]. According to these criteria insomnia is defined as the experience of problems to fall asleep, to maintain sleep or to suffer from early morning awakening. These sleep symptoms have to be coupled with daytime impairments, like decreased attention or problems in concentration. These symptoms have to occur at least three times a week over a period of at least 3 months in order to be diagnosed as insomnia disorder. If the insomniac symptoms are clearly due to another medical/ mental disorder/sleep disorder or substance use, they are not diagnosed separately. However, in most cases, insomnia may have occurred before the medical or mental disorder, or may persist beyond the medical or mental disorder even when the other disorder has been treated successfully. In these cases, diagnostic comorbidity is preferred to single diagnoses. Insomnia as a symptom occurs very frequently (>50% of the population in a year) and in many cases will disappear, for example after the cessation of an acute stressor [ 51 , 52 ]. It is assumed that ~10% of the population in industrialized countries suffer from chronic insomnia [ 53 ]. Recent treatment guidelines [ 54 , 55 , 56 ] have summarized that insomnia is associated with high costs for the health care system and also for a high degree of suffering for afflicted individuals. Treatment constitutes of benzodiazepines, benzodiazepine receptor agonists, ramelteon, suvorexant and other substances, which are administered off-label like sedating antidepressants—an interesting development further underlining the close relationships between insomnia and depression. As of now, the first-line treatment of insomnia according to current guidelines is cognitive-behavioral therapy for insomnia (CBT-I) [ 54 , 55 , 56 ]. Importantly, the diagnosis of insomnia—as with all other mental disorders—is based on the subjective experience of the afflicted individual and not on PSG defined criteria.
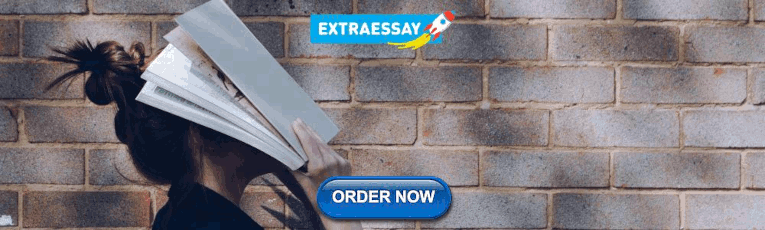
Insomnia and hyperarousal
The idea that insomnia might be due to “hyperarousal” is a very old one and can frequently be found already in the medical literature of the nineteenth century (“overexcitation of the nerves”) or even earlier. We have documented [ 51 , 52 , 57 , 58 ] that the hyperarousal hypothesis offers an explanation into the origin of insomnia. The literature looking at psychophysiological variables of insomnia can be summarized as follows: parameters of the autonomous nervous system, the endocrine system, the neuroinflammatory system and neurophysiological indices clearly show that patients who suffer from insomnia show increased indices of hyperarousal, as reflected by altered heart rate, increased cortisol output, and an increase in fast waves as documented by increased amounts of beta waves measured by the sleep EEG [ 59 ]. On the psychological level numerous studies have demonstrated that hyperarousal and hyperarousability, as measured by questionnaires, is something very typical for patients with insomnia, which they also experience directly on a subjective level (e.g., racing thoughts whenever trying to sleep). The hyperarousal model of insomnia can be easily linked to neurobiological models of sleep and sleep regulation such as the flip-flop switch model of sleep regulation formulated by Saper et al. [ 60 ] (see also the first article in this volume and section “The flip-flop switch model and the role of the orexinergic system”).
Insomnia as a predictor of depression and suicidality
The renowned interest into the relationships between sleep and circadian rhythms and depression is mainly due to studies that have shown that insomnia/insomniac symptoms are independent predictors for depressive disorders [ 61 , 62 , 63 , 64 ] and suicidal ideation/ suicide and suicide attempts [ 65 , 66 , 67 , 68 ]. These epidemiologically based data have led to great interest in sleep continuity changes in the context of depression and other mental disorders. Thus, the quest for specific biological markers was given up in lieu of focussing on transdiagnostic overarching markers/mechanisms, which can be elucidated by subjective self-report, rendering research and clinical applicability easier and economic.
Sleep and insomnia: bi-directional relationships with depression
Given the fact, that on the one hand, almost all depressed patients display some kind of sleep alteration, and that on the other hand especially insomnia alone conveys an increased risk for depression and suicidality, the sleep-depression relationship needs to be conceptualized as bi-directional. Several observations converge with this view: When comparing etiological and pathophysiological explanations for insomnia and depression, a clear overlap is present—both conditions have been shown to be triggered by psychosocial stressors. Hyperarousal being regarded a psychological and a biological factor is present in both conditions. When considering treatment modalities, sedating antidepressants can be used for both conditions and CBT-I, the most effective insomnia treatment, has—to some extent—been derived from classical CBT for depression. On the other hand treating insomnia early has the potential to prevent or reduce depressive symptoms as promising preliminary data indicate. We suggest to acknowledge that both disorders can occur independently of each other, as evidenced by the fact that many patients with chronic insomnia never develop depression. Equally, insomnia might indicate a first step towards onset of psychopathology, sharing some underlying (epi-) genetic, personality and neurobiological changes typical for depression. Whether or not depression develops might depend on additional environmental triggers, such as psychosocial stress load, lifestyle, coping mechanisms and early preventative treatments. Given the fact that by and large only a minority of patients with insomnia is sufficiently treated (with CBT-I), there seems to be ample room to test the hypothesis whether stringent and early insomnia treatment might reduce the risk for becoming depressed in the long run.
Treatments of insomnia—a chance for prevention of depression?
As mentioned before, presently Cognitive-Behavioral Treatment for Insomnia (CBT-I) is acknowledged to be the first-line treatment for insomnia [ 54 , 55 , 56 , 69 ]—thus opening the possibility for large-scale studies to test whether early and adequate treatment of insomnia may prevent psychiatric sequelae, i.e., depression or psychosis. First studies targeting this issue showed that online delivered CBT-I seems to be able to reduce insomnia and depression scores in subclinically depressed patients with insomnia [ 70 ]. In a similar vein, advances in the field of chronobiology have led to a renaissance of chronotherapeutic approaches for mental disorders [ 71 , 72 , 73 ]. These strategies are useful non-pharmacological preventive implementations into everyday lifestyle—regular sleep-wake rhythmicity, season-adapted daily food and physical activity, day structure, correct light exposure at the “right” time in indoor lighting—all strategies showing depression-preventive properties, which started to be empirically tested and confirmed but need replication.
Insomnia as a transdiagnostic symptom/ syndrome for psychopathology
A further boost for the field came from DSM-5 [ 50 ] with the establishment of the category of insomnia disorder, thus ascribing independent value to this symptom complex instead of considering it mainly a symptom of any kind of mental disorder. Still, in DSM-5, separation of condition by categories is current practise but clinical research inspired by RDoC (Research Domain oriented Criteria; www.nimh.nih.gov ) is supporting a dimensional approach using constructs and domains to understand pathophysiology. RDoC has suggested a major domain named “arousal and regulatory systems” with the constructs “arousal”, “circadian rhythms” and “sleep-wakefulness” with a detailed listing of areas of interest for research from the level of molecules to circuits, behavior and paradigms. Two recently published meta-analysis on PSG derived sleep variables in insomnia and all different types of mental disorders [ 13 , 74 ] support this concept and stress a transdiagnostic approach of sleep continuity disturbances/ insomnia towards mental illness. Instead of adhering to an approach, which sought to identify disease-relevant mechanisms through identifying biological markers for specific mental disorders, we postulate that (i) sleep and circadian rhythm disturbances occur independently of and predict/coincide with affective disorders, (ii) clinical psychopathological syndromes do not necessarily reflect homogenous pathophysiological origin, (iii) neuropsychiatric syndromes like depression and sleep/circadian disturbances are linked through common mechanistic origins [ 58 , 75 ].
Neurobiology of sleep and circadian rhythms—relevance for depression
This chapter highlights recent developments in the field with emphasis on the so-called “flip-flop” switch model, the neuroplasticity hypothesis of sleep and depression, and neural biological timekeeping being pivotal in generating rhythmic behavior.
The flip-flop switch model and the role of the orexinergic system
Saper et al. [ 60 , 76 ] have developed neurobiological models of sleep-wake and REM-NREM (non-rapid eye movement sleep) regulation (details see Fig. 4 ; see also the first article in this issue of NPPR) proposing bistable switch mechanisms between wake and sleep-promoting cell populations, as well as REM and NREM promoting clusters. Wakefulness is governed by a network of cell groups in the hypothalamus (including orexinergic neurons), basal forebrain, and brain stem, which activate the thalamus and the cerebral cortex. These wake-promoting centers include but extend beyond the cell groups in the reticular formation of the brain stem originally described as the ARAS (ascending reticular activating system) by Moruzzi and Magoun [ 77 ]. As the main sleep-promoting center, the VLPO (ventrolateral preoptic nucleus) is primarily active during sleep, gives output to all major wake-promoting centers in the hypothalamus and brain stem that participate in arousal and reduces their activity using the inhibitory neurotransmitters galanin and GABA (gamma-aminobutyric acid). The VLPO also receives afferent signals from each of the major monoaminergic systems and its neurons are inhibited by noradrenaline and serotonin. Saper and colleagues postulate that this mutual inhibitory circuit constitutes a “flip-flop switch” with sharp state transitions between wake and sleep. The state of this intricate network is governed by circadian and homeostatic processes and exerts sleep-wake regulation in animals and in humans. It may be speculated that a dysfunctional “key switch” plays a major role in the pathogenesis of primary insomnia. An imbalance between sleep-promoting areas in the Central Nervous System (CNS) (i.e., the VLPO, neurotransmitter: GABA) and arousal-inducing neurons (among others orexin neurons in the lateral hypothalamus) with a relative overactivity of the orexin system or a hypofunction of the VLPO might create an unphysiological hybrid state between sleep and wakefulness. This offers a possible neurobiological model for insomnia (see section “Circuit-based interrogation of a shared neurobiological pathogenesis of insomnia and depression using animal models”). We speculatively assume that an overactivation of the arousal system is present both in insomnia and in depression with hyperarousal explaining the close association between both conditions. Considering the role of the orexin system in regulating both the arousal and the affective system, it could be possible that enhanced production of orexin related to psychophysiological hyperarousal increases the risk of reacting with enhanced emotional activation to stressful situations.
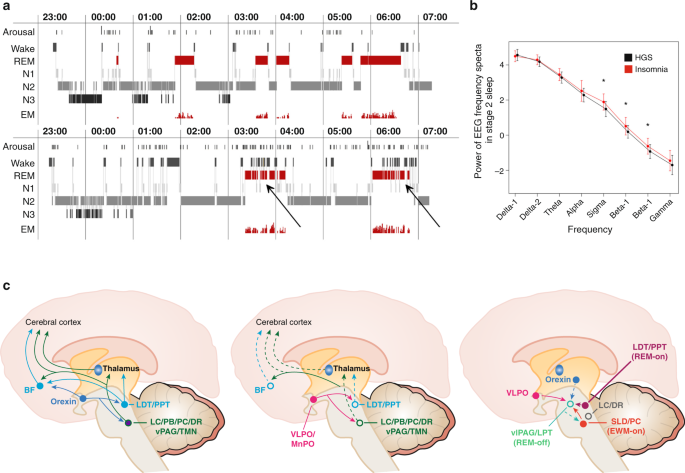
This figure displays the relationships between normal and insomniac sleep, spectral analysis of sleep EEG and neuroanatomical structures as delineated from the “flip-flop” switch model. a Upper panel—healthy sleeper; lower panel—insomniac sleeper: It is shown that there is no grossly disturbed macrostructure of Non-REM-REM sleep cycling in insomniac patients, but an altered microstructure with many brief awakenings and arousals in insomnia especially in REM sleep (see arrows). b Here shown are spectral analytic data from healthy good sleepers (HGS) and people with insomnia, reflecting a significant increase in fast waves especially in the sigma and beta range. c Anatomical structures and pathways involved in the regulation of wake (left), Non-REM (middle), and REM sleep (right) according to “flip-flop” switch model (see text). Panels a and b of the figure are taken from ref. [ 53 ]—permission granted. Panel c is based on refs [ 60 , 76 , 193 ]—permission granted
The orexinergic system [ 78 , 79 , 80 ] also orchestrates other depression-related factors such as behavioral and neuroendocrine responses to stress, reward-seeking behaviors, energy homeostasis, learning and memory. The orexin system directly innervates and excites noradrenergic, dopaminergic, serotonergic, histaminergic and cholinergic neurons. It also has a major role in modulating the release of glutamate and other amino acid transmitters and in enhancing hippocampal neurogenesis. Contradicting our line of reasoning, it has to be mentioned that narcolepsy, a debilitating disorder of excessive hypersomnolence (coupled with the frequent occurrence of shortened REM latencies) has been shown to be related to orexin deficiency [ 81 ].
Sleep, depression, and neuroplasticity
The synaptic plasticity hypothesis of major depression posits that alterations of synaptic plasticity represent a final common pathway for the clinical manifestations of the disorder. The hypothesis is based on animal models of depression [ 40 ], postmortem studies in humans and on non-invasive indices of plasticity in humans [ 41 ]. Of particular interest, sleep has been identified as a potential modulator of synaptic plasticity [ 82 ]. Current models emphasize that sleep might promote the strengthening of newly induced information-bearing synaptic connections, while—through down selection of less relevant synapses—keeping overall network function stable [ 42 , 83 ].
In particular, two links between sleep and depression are discussed. First, chronic sleep disruptions in the form of insomnia might disrupt synaptic plasticity and neural network function. Particularly, a dorsal executive network that includes the hippocampus and the prefrontal cortex appear to be particularly sensitivity to sleep loss, resulting in deficits such as attenuated hippocampus-dependent declarative memory formation in chronic insomnia. A ventral emotional network, which includes the amygdala, might be more resilient (for instance indexed by emotional fear conditioning) and might only decompensate with chronic severe sleep disruptions [ 84 ], relating to the clinical trajectory from cognitive to emotional complaints with chronic sleep disruptions. Second, it was recently proposed that therapeutic sleep deprivation—through a homeostatic, wake-related increase in overall synaptic strength—transiently shifts initially deficient net synaptic strength in patients with depression into a more favorable window of associative synaptic plasticity, related network function and behavior [ 44 ] (see also section “Biological timing and sleep deprivation in depression”). Proposed, but not sufficiently confirmed neural mechanisms include a BNDF, p11, Homer1a, and AMPA receptor cascade of rapid antidepressant treatment, potentially shared with the rapid antidepressant mechanism of ketamine [ 85 ]. While the level of evidence remains moderate and numerous questions open, such as specificity, these lines of research allow for deriving testable hypotheses, for instance on the effects of selective modulation of sleep slow waves through auditory or current stimulation, and potentially for the development of new, rapid acting antidepressive treatments.
Chronobiological timekeeping approaches
Compelling evidence suggests that mood disorders arise in part from alterations in the biological timekeeping system, triggered from within the body or from the body’s response to changes in the external environment. Each part of the human body has developed timekeeping mechanisms on every level, from molecular, cellular, neuronal, endocrine up to organ level, affecting the entire body. The timekeeping system is not hierarchical but has a multi-oscillatory structure [ 86 ]. Individuals with depression are likely to have internal oscillatory systems out of sync or dampened, but it is difficult to pinpoint the rhythms’ phase-relationships at every level. A classical psychopathological feature in this context is the diurnal mood variation [e.g., ref. [ 38 ]], observed in many patients with depression, which is indicated as a “morning low” in mood and a spontaneous alleviation of mood in the afternoon/ evening. Other well-known rhythmic features include seasonal modulation of onset of depression or rapid-cycling phenomena with strict periodicities [ 87 ]. Disrupting the body’s stable time patterns by shift work and jet lag can worsen or cause changes in mood. Various hypotheses have been suggested to explain these altered biological rhythms in affective disorders. One concerns disruption within the master pacemaker, the suprachiasmatic nuclei (SCN), causing mood disturbances [ 88 ], whereas another suggests that light directly from the retina and bypassing the SCN, targets brain regions to control mood [ 89 ]. Endogenous processes oscillate with an approximate cycle of 24 h, in adaption to the solar day-night cycle, and hence rhythms with this length are named ‘circadian’’ rhythms. However, many other functions have a more frequent activity pattern, called ‘ultradian’’ rhythms, for example the NREM-REM sleep cycles. At the behavioral level, human sleep-wake pattern displays circadian rhythmicity, while our food-intake displays an ultradian rhythmicity. Both are under the influence of the light–dark cycle whereby day light plays a crucial role in setting the activity of the SCN to the local environment. At the cellular level, circadian rhythms are generated by a molecular clockwork that consists of multiple transcriptional/translational feedback loops [ 86 ]. Nearly all tissues express circadian genes (e.g., Bmal1, Clock, Per , and Cry ), which heterodimerize in specific combinations, and regulate the expression of many clock-controlled genes, as well as their own transcription in a feedback fashion. These oscillatory processes ensure cellular timekeeping within tissues, while the SCN generates self-sustaining circadian oscillations, which ensure the synchronization of bodily rhythms. Some studies from individuals with depression found abnormal signaling in SCN cells to correlate with disease duration [ 90 , 91 ], while others reported altered nitric oxide signaling in the SCN [ 92 ], as well as in response to changes in the light–dark cycle [ 93 ] and nitric oxide has also been implicated in mood regulation [ 94 ]. Hence this substance may affect mood and circadian regulation independently and synergistically. While receiving and integrating information, the SCN maintains a functional circuit with the paraventricular nucleus (PVN), which is important for the control of pituitary hormones and melatonin secretion from the pineal gland [ 95 ]. Disturbances in the functioning of this circuit can potentially explain hormonal alterations associated with depression. Another structure with a direct connection to the SCN is the lateral habenula, which couples dopaminergic and serotonergic systems [ 96 ] and which is receptive to deep brain stimulation to treat depression [ 97 , 98 , 99 ]. The lateral habenula not only exhibits intrinsic neuronal oscillates but also responds to retinal light exposure directly [ 100 ] and by bringing it all together, it could be an important interface between retinal light, the pacemaker’s rhythms and multiple monoaminergic brain regions that control mood and motivational behaviors, stress and inflammatory systems, reward circuits, arousal and sleep. Melatonin secretion in the pineal is indirectly regulated by retinal light exposure involving the SCN and the PVN in a multi-synaptic pathway, and its secretion span feedbacks time-of-day information to MT1 and MT2 receptors, which are widespread in the brain, including the SCN, and in peripheral tissue [ 101 ]. Sleep deprivation (SD) with “lights on” suppresses melatonin synthesis in the pineal, dampens the neuronal firing rate in the SCN and attenuates light-induced phase-shifting. Moreover, SD changes circadian gene expression in brain regions involved in mood regulation, but not the SCN, while arousal due to SD when supposed to sleep, leads to an increased level of serotonin in the SCN [ 102 ].
Given these diverse relationships, it seems reasonable to test combinations of chronotherapeutic interventions on a larger level to derive an estimate of their clinical usefulness.
Synthesis—a model of the bi-directional relationships between sleep and depression
A comprehensive neuropsychobiological model linking the fields of insomnia and depression research is summarized in Fig. 5 .
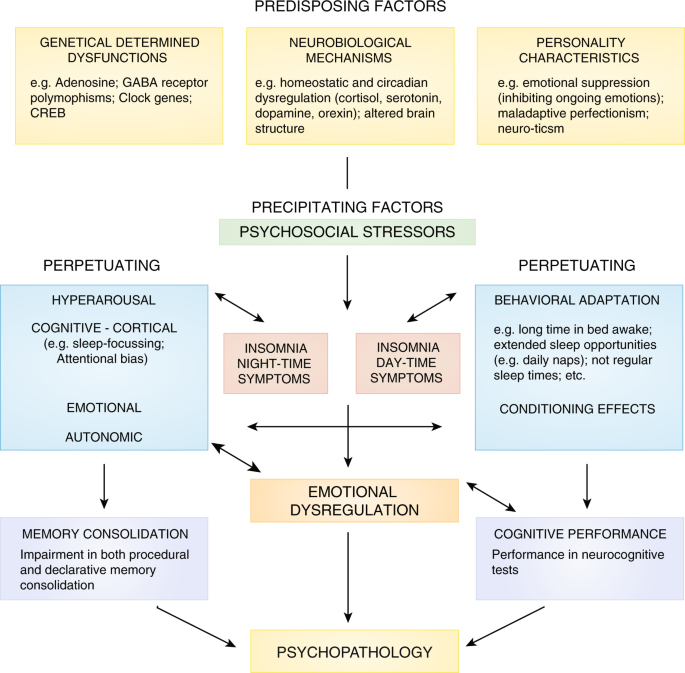
Comprehensive insomnia model (see text)—authors´ own conception
This neuropsychobiological model integrates different strands of research from the fields of genetics, neurobiology, personality research, psychophysiology and cognitive-behavioral research to provide a comprehensive understanding of the mechanisms involved in the etiology and pathophysiology of insomnia and its role for the development of psychopathology, especially depression. The basic structure of this model is taken from the 3P model of insomnia formulated by Spielman et al. [ 103 ]. The 3P´s stand for: P redisposing, P recipitating, and P erpetuating factors.
Predisposing factors stem from the fields of genetics, neurobiology and personality.
In short [ 104 ] genetic and epigenetic factors [ 105 ] have been proven to be involved in the etiology and pathophysiology of insomnia by family and twin studies. Two GWAS studies [ 106 , 107 ] pointed to an involvement of MEIS1, which is also associated with the restless legs syndrome. Lane et al. [ 108 ] applied Mendelian randomization analysis and concluded that reliable evidence for a possible causal link between insomnia symptoms, coronary artery disease, depressive symptoms and well-being has been established.
Neurobiological mechanisms, like the flip-flop switch model (see section “The flip-flop switch model and the role of the orexinergic system”), the neuroplasticity hypothesis of sleep and depression (see section “Sleep, depression, and neuroplasticity”) and biological timekeeping mechanisms (see section “Chronobiological timekeeping approaches”) have to be taken into account when constructing a bridge between sleep, insomnia and depression on the cellular level.
With respect to personality variables as predisposing factors, sleep reactivity, the tendency to exhibit pronounced albeit transient sleep disturbance in response to stressful events, has been shown to be a major risk factor for chronic insomnia [ 109 ]. Moreover, several personality traits have been linked to insomnia, including neuroticism, perfectionism, sensitivity to anxiety symptoms and the tendency to internalize problems [ 110 ], some of which also seem to be depression-related.
Precipitating factors are significant life events, which facilitate the onset of acute episodes of insomnia. The most frequently reported triggers of acute episodes of insomnia are stressful life events that are related to threat of security to family, health, and work/school living, that lead to a negative emotional valence [ 111 , 112 ]. Additionally, experimental work using stress-induction paradigms clearly showed that acute stress has a negative effect on sleep initiation and sleep maintenance. Needless to say that life events have also been linked with depression.
Perpetuating factors: With respect to hyperarousal, we extended the hyperarousal concept (see section “Insomnia and hyperarousal”) to a more specific hypothesis of REM sleep instability as a possible pathway to insomnia and depression [ 57 ]. The concept is based on PSG findings showing that primary insomnia is characterized by a decreased amount of REM sleep and increased EEG arousals during REM sleep [ 113 ]. REM sleep regulation is governed by a unique neuronal pattern, which requires a delicate balance between arousing and de-arousing brain activities to sustain this highly aroused sleep brain-state accompanied by muscle atonia. This activity is dominated by cholinergic input to higher brain areas and an inhibition of noradrenergic, serotonergic and orexinergic output. The hypothesis of “REM instability” suggests that a generally increased arousal level leads to a modest REM sleep reduction and fragmentation in primary insomnia. This in turn results in altered perception of mental processes taking place during the REM sleep state: instead of experiencing dreams, patients with insomnia, due to the nature of their pre-sleep concerns, emotions and cognitions, might experience their REM sleep mentation as more wake-like and centered on their main concern, i.e., insomnia [ 114 ]. With respect to the relationship with depression, we propose that a chronic fragmentation of REM sleep in insomnia might interfere with basal processes of emotion regulation. With persistence of the disorder and hence REM sleep reduction and fragmentation, at some point a REM sleep rebound (leading to shortened REM latency and increased REM density) and a more stable pattern of emotional reactivity characterized by enhanced responses to negative stimuli (both subjectively and objectively measured) may occur, which may facilitate the development of a depressive episode.
Behavioral perpetuating factors include prolonged time in bed, an irregular sleep-wake schedule and daytime napping [ 103 ]. Many patients with insomnia use excessive time in bed and daytime napping as compensatory strategies against their perceived sleep loss. However, these strategies lead to more-pronounced difficulties in sleep-onset and sleep-maintenance [ 103 ]. Classical conditioning has also been suggested as an important perpetuating factor in insomnia [ 115 ]. In particular, it has been suggested that the bed and the bedroom environment of patients with insomnia become conditioned to arousal and anxiety during an acute episode of insomnia leading to sleep initiation and maintenance problems even after the removal of the initial stressor. In addition to this, a large body of evidence shows that worry and rumination are involved in the maintenance of insomnia [ 116 ]. These unproductive thought processes are believed to be associated with a level of physiological arousal that is incompatible with sleep initiation and maintenance. Moreover, self-report studies on emotions in insomnia showed an increased experience of negative emotions in general and specifically at bedtime [ 117 ].
What is known about the relationships between sleep, insomnia and the regulation of emotion? Experimental data suggest that people with insomnia report more negative emotions both in general and close to sleep time as compared to good sleepers [ 118 ]. Very recent results from negative emotional stimulation show that this response is correlated with a reduced activity of the amygdala and with a moderate level of arousal when compared to good sleepers [ 119 ]. In summary, altered emotional responses, both subjective and physiological, have been shown for insomnia. The sensitivity of emotion regulation in insomnia may explain the close association between insomnia and depression. Considering possible biological mechanisms involved in the link between sleep alterations, insomnia, emotion regulation, and mood symptoms, orexin neurons have been found to be related to both sleep-wake regulation as orexin is inhibited during sleep by GABA and galaninergic neurons from the ventrolateral preoptic nucleus (VLPO) (see section “The flip-flop switch model and the role of the orexinergic system”) and affective processes. Consistently, efferent pathways from the amygdala to the lateral hypothalamic area (LHA) have been identified and orexin production has been shown to be activated by emotional events, especially related to emotional stress [ 120 , 121 ].
Present management of sleep/ circadian disorders in depression
Subjectively reported sleep disturbances typically manifest in various forms, such as difficulties falling asleep and maintaining sleep, waking up too early and feeling worn, fatigued and sleepy during the day. Although these symptoms are described by DSM-5 as insomnia disorder, they are rarely investigated properly by general practitioners or mental health professionals—unfortunately at present it is far more probable that both insomnia and symptoms of circadian dysfunction are still more or less viewed as symptoms of an underlying mental disorder, which will, at best, remit when the “primary” (=underlying) disorder is properly treated or, at worst, treated with the wrong drugs. A main reason for this is that there is a serious lack in the knowledge about basic sleep and chronomedicine across fields in the medical professions, maybe because these disturbances are transdiagnostic. Insofar, there still is a strong need for further education of health professionals, in particular in psychiatry (hospital and community doctors, nurses, health advisors), in the domain of sleep and chronomedicine to enable them to recognize, properly diagnose and treat individuals with sleep problems [ 122 ]. Specific evidence-based treatment strategies incorporating chronomedicine (right drug, right dose, right time) for these disorders are available and might offer not only to improve the underlying sleep/circadian disorder but concomitantly ameliorate the outcome and course of mental disorders compared to standard psychiatric treatment.
Cognitive behavioral therapy for insomnia (CBT-I)
For non-pharmacological insomnia treatment the most extensive data basis exists now for CBT-I [ 69 ]. CBT-I has been primarily investigated in primary insomnia and studies are just gathering momentum to evaluate its efficacy and effectiveness in co-morbid insomnia. Most recent guidelines for insomnia treatment from the US and Europe [ 54 , 55 , 56 ] conclude that CBT-I is the first-line treatment, whereas recommendations for all investigated hypnotic drugs are not stronger than “weak”, and mostly for the short-term treatment (3–4 weeks; AASM, 2017) [ 123 ]. The AASM stresses that these rather low rankings for all existing hypnotics, especially benzodiazepines, benzodiazepine receptor agonists etc. reflect the fact that the Grade system (used to make the recommendations) downgrades the quality of evidence if the funding source is a pharmacological company. Furthermore, the AASM states that a weak recommendation should not be construed as an indication of ineffectiveness. The ultimate decision to prescribe sleep medication should be based on the clinician´s decision reflecting all individual circumstances, the patient´s shared consent and the availability of alternative treatment options in a given clinical setting. All of these guidelines [ 54 , 55 , 56 , 123 ] were based on thorough analyses of the relevant literature. Thus, either published meta-analyses (based on randomized clinical trials/ RCT)) or single RCTs were considered to evaluate the evidence. Insofar, the above-mentioned guidelines can be considered as based on the highest levels of presently available evidence. These new guidelines also indicate that CBT-I has significant long-term effects beyond the acute treatment phase, in that respect being superior to pharmacological treatment for primary insomnia. CBT-I encompasses relaxation techniques, sleep hygiene, stimulus control, sleep restriction and cognitive techniques to reduce nocturnal ruminations [ 124 ]. In psychological models of insomnia like for example the 3P Model [ 103 ], the model of Morin [ 52 ] or the cognitive models of Harvey and Espie [ 125 , 126 ] components of CBT-I can be easily matched to the psychological components of the pathophysiology of insomnia. It is also of interest that the 2-process model of sleep-wake regulation and the concept of sleep propensity/sleep pressure [ 25 ] have been at the core of informing behavioral strategies for insomnia, i.e., sleep restriction: this strategy is derived from the clinical observation that patients with chronic insomnia prolong time in bed to achieve more sleep—a behavior, which unfortunately perpetuates insomnia instead of relieving it. By shortening time in bed (as suggested by sleep restriction), in contrast, the homeostatic sleep drive is stimulated and subsequent sleep quality improved [ 127 , 128 ]. Considering sleep restriction as a kind of partial sleep deprivation, it is interesting to note that total sleep deprivation on its own is a rapid, albeit transient, treatment modality in depression (see section “Biological timing and sleep deprivation in depression”).
Hypnotics, chronobiotics, antidepressants
Regarding prescription drugs such as benzodiazepines (BZ)/benzodiazepine receptor agonists (BZRA), melatoninergic agents, suvorexant, sedating antidepressants and atypical antipsychotics are used in the wider field of insomnia and for sleep problems within the context of depressive disorders. Evidence from clinical studies is strong for effective short-term administration (3-4 weeks) of BZ/BZRA for insomnia [ 55 , 56 , 123 ]. Doubtlessly, hypnotic agents like BZ, BZRA, or suvorexant will produce stable positive effects on patient´s sleep over a window of 3–4 weeks; however, given the fact that practically all performed studies were sponsored by the pharmaceutical industry, overall recommendations for example by the AASM [ 123 ] could not be beyond “weak”. Many patients afflicted with insomnia suffer chronically and there is doubt for effective long-term treatment beyond four weeks with these drugs. A major concern with long-term BZ/BZRA treatment is the issue of adverse events (e.g., traffic safety, mnestic effects, paradoxical behavior during the night, intoxication, falls in the elderly etc [ 129 , 130 , 131 ]) and the development of tolerance/rebound and abuse/dependency [ 132 , 133 , 134 ]. These and related issues concerning the risks of BZ/ BZRA for increased mortality [ 135 , 136 ] are discussed controversially, because other studies [ 137 , 138 ] highlighted the likely role of confounding factors when relating risk of cancer or mortality to BZ/BZRA intake. Nevertheless, especially the issue of potential risks concerning abuse or dependency probably are responsible for the decision that treatment of insomnia with these agents beyond 3–4 weeks is not endorsed in Europe by drug regulating authorities. A different stance has been taken by the FDA (US American Food and drug administration) in recent years granting long-term use for all newly admitted hypnotics since 2004 (e.g., eszopiclone).
Melatoninergic agents like ramelteon [ 139 ] have been admitted to the US market. In Europe, a prolonged release formulation of melatonin Circadin, can be prescribed for long-term (3 months) treatment of insomnia in the over 55-years-old [EMEA: http://www.emea.europa.eu/humandocs/PDFs/EPAR/circadin/H-695-de1.pdf ]. The benefit of melatonin treatments with respect to circadian rhythm disturbances, like jet lag and phase delay [ 140 ] has been proven. However, the evidence for melatonin being beneficial in the treatment of insomnia is not very convincing [ 141 , 142 , 143 , 144 , 145 ].
Sedating antidepressants (SED; e.g., amitriptyline, doxepin, trimipramine, trazodone, mirtazapine, agomelatine or atypical antipsychotics (AP; quetiapine, olanzapine) are frequently used for symptoms of sleep disturbances in patients with mental disorders, i.e., major depression or bipolar disorder or schizophrenia [ 146 ]. They are not indicated for the treatment of insomnia without mental comorbidity. However, recent pharmaco-epidemiological studies revealed that in the US (for example trazodone) or in Europe (for example trimipramine, mirtazapine) sedating antidepressants are increasingly used in that field [ 147 , 148 ]. The efficacy and effectiveness of SED and AP to treat major mental disorders is undisputed—however, there still is a lack of data from large controlled trials, apart from a few pilot studies [ 149 , 150 , 151 ] and a recent meta-analysis [ 152 ], demonstrating clearly that these compounds are superior to placebo when addressing insomnia alone and sleep disturbances in mental disorders. Interestingly, doxepin, one of the “oldest” available SEDs, has been shown to be effective at very low doses in primary insomnia [ 153 ] and thus gained approval by US Food and Drug Administration. With respect to AP even less evidence is available—studies are available investigating the effects of AP on sleep in schizophrenia [ 154 ] and in insomnia [ 155 , 156 ]. At present, it is safe to conclude that most AP are clinically useful to combat sleep problems in a subset of patients with schizophrenia, but not for insomnia without mental comorbidity.
Behavioral chronotherapy and chronopharmacology
Behavioral chronotherapeutic approaches encompass bright light therapy, dark therapy, dawn simulation, sleep-wake manipulations (sleep deprivation, sleep phase advance/delay), often in combination with precisely personalized timed low-dose fast-release melatonin agents, as well as social rhythm therapy [ 72 , 73 ]. Application of bright light, usually for periods of 30–60 min at an intensity of 5000–10.000 lux in the morning (dependent on indication) or the use of a dawn simulator with a slow increase in light over 30 min around wake-time, is used therapeutically in circadian sleep-wake rhythm disorders, such as phase advance/delay, seasonal affective disorders (SAD), major depression and geriatric disorders (e.g., dementia). Dark therapy is successfully used to prevent manic phases in patients with bipolar disorder [ 157 ]. Sleep-wake manipulations include total and partial sleep deprivation (SD) and phase shifts of the sleep phase (sleep phase advance therapy). This type of manipulation is dominantly used in patients with major depression and especially those suffering from melancholia displaying clinical features like diurnal mood variations. Timed light exposure and type of light should be considered as important during sleep deprivation since light affects the circadian timing system. Wu and Bunney [ 34 ] published a systematic review on SD demonstrating that total SD produces a clinically significant, albeit short-lived, remission in mood in as many as 60–70% of patients with severe depression. Social rhythm therapy (SRT) aims at affective disorders, especially bipolar depression. In combination with specific psychotherapy (interpersonal therapy) and the administration of mood stabilizers (e.g., lithium), SRT aims at reducing the impact of stressful life events on the clinical course of the disorder by addressing issues of lifestyle regularity [ 158 , 159 ]. With respect to circadian disturbances, studies have assessed melatonin’s efficacy in the visually impaired (diminished light input to the pacemaker), jet lag syndrome, shift work, phase advance/ delay, depression and neurodevelopmental disorders like autism with promising results [ 140 , 160 , 161 , 162 , 163 ].
Another very relevant aspect of chronomedicine is Chronopharmacology, the timing of drug intake. Antidepressants in particular involve a number of neurotransmitters that are acting directly on the SCN or in circuits, which are regulated by the timekeeping system, for example the SCN has serotonin receptors and lithium is known to prolong the circadian period.
Unfortunately, up to now no data are available how frequent chronotherapeutic approaches are used for the treatment of circadian and other disorders—this is in contrast to prescribed medications and psychotherapeutic treatments, where usually sales figures or statistics derived from national health care systems can give at least a rough indication how frequently a certain intervention is applied. This is in part due to the fact that for example an intervention like sleep deprivation or light therapy in a depressed patient applied in a hospital will produce no tangible costs to figure in a statistic—thus apart from enhancing awareness for circadian/chronobiological disorders it will also be a challenge to collect data on the specific treatment modalities in clinical care to get an impression of their effectiveness in routine clinical care.
Non-invasive brain stimulation
Recent work suggests that arousal and sleep can be modulated by targeting a cortico-thalamic top-down pathway of sleep-wake regulation through different types of non-invasive brain stimulation (NIBS), including transcranial magnetic stimulation (TMS), transcranial current stimulation (tCS) or sensory stimulation, such as auditory stimulation [ 164 ]. Of note, patients in the diagnostic category of major depression might suffer from different sleep complaints along the domain of hyper- (insomnia) and hypoarousal (prolonged sleep duration). This domain may be suitable for structuring future NIBS research and treatment development, because it can be characterized on different levels, including the genetic, molecular, neurocircuitry and behavioral level. Of particular interest, it might be possible to identify more fine-graded alterations in individual patients, such as alterations of sleep slow waves, sleep spindles or local aspects of sleep that might be targeted with specific NIBS techniques. To date, several NIBS approaches have been used to modify sleep: Transcranial magnetic stimulation (TMS) uses a magnetic field, which modulates cortical activity. For instance, specific EEG patterns such as sleep slow waves and spindles can be triggered using this technique [ 45 , 46 ]. However, the TMS setting during sleep is demanding and limited to specific research questions. Transcranial current stimulation (tCS) can induce local shifts in cortical excitability [ 165 ] and has the potential to affect distinct aspects of sleep. For instance, anodal tDCS during slow wave sleep improved declarative memory [ 166 ]. Moreover, anodal tDCS can reduce total sleep time by ~25 min in healthy humans [ 167 ], but does not modify sleep in patients with insomnia, indicative of brain-state-dependent effects of the stimulation protocol [ 168 ]. While tDSC is easily applicable, the clinical relevance of the observed effects remains unclear. Auditory stimulation applied in a brain-state-informed protocol (auditory closed-loop stimulation) can enhance sleep slow waves and sleep-related memory consolidation [ 45 ] or selectively suppress slow waves and modulate neurophysiological measures of neuroplasticity [ 46 ]. These approaches bear the potential to target distinct aspects of sleep, to increase our mechanistic understanding and, potentially, to develop new sleep-based treatments for depression. Yet currently, no clinically relevant sleep-based interventions in this area are known.
Key directions for future research and clinical practice
Establish cbt-i as preventive strategy for depression.
Unfortunately, apart from research studies, CBT-I up to now has not been established in routine clinical practice or care in the US or European countries. In order to overcome this problem a stepped-care approach has been suggested by Espie [ 169 ]. According to this model, in a first step self-help approaches in bookform or internet-based programs can be used by afflicted individuals with mild to moderate symptoms [ 170 ]. In a next step, community-based approaches involving group courses given by trained nurses would reach larger numbers of patients face-to-face [ 171 ]. Contact with a qualified clinical psychologist/ psychiatrist or sleep specialist would be reserved for the most severe cases to administer state of the art CBT-I or abbreviated forms of this treatment [ 172 , 173 ]. Very recently, a large- scale study with digital cognitive-behavioral therapy for insomnia found sustained effects of improved psychological well-being and sleep-related quality of life resulting from an 8-week sleep-hygiene education program [ 174 ]. This provides first evidence that psychoeducation via web and/or mobile channels in addition to usual treatment is an easy-to-use and effective element in a stepped-care approach for insomnia.
Furthermore, the central question to address will be the efficacy and effectiveness of CBT-I for insomnia co-morbid with mental disorders—there is promising evidence now even in the form of meta-analysis [ 175 , 176 ]. Thus, larger-scale longitudinal studies are needed to prove that CBT-I adjuvant to standard psycho-and pharmacotherapy in different populations of psychiatric patients not only positively influences insomniac symptoms but beyond improves the general outcome of the disorder. In the same vein, the often-quoted assumption that insomnia treatment may prevent psychiatric sequelae in chronic insomniacs [ 62 ] needs to be proven—data from the “GoodNight” study [ 177 ] point to a first empirical confirmation of this assumption [ 70 ].
Integrate chronobiological interventions into the armamentarium of antidepressant treatments
It represents a timely and urgently needed step to translate the impressive basic science knowledge on circadian rhythmicity to the clinic. This translation needs to address the following points: (i) provide guidance for a clinically useful estimation of the individual circadian phase (e.g., through the wake-up time or mid sleep phase), (ii) provide recommendations for the application of melatonin and light with regard to the individual phase response curve, (iii) provide recommendations on the duration, monitoring and optimization of the treatment (e.g., phase-shift during therapy), (iv) test for effects on circadian rhythms, sleep, mental disorders and broader health outcomes. Although the points outlined above appear trivial referred to the deciphering of the molecular mechanisms of circadian rhythms, there is to date, to our knowledge, no practical recommendation for implementing circadian interventions into broader clinical practice,
Novel molecules for depression and sleep disturbances
As can be deduced from the section on the regulation of sleep and its neuropharmacology novel pharmaceutics may target noradrenergic, serotonergic, histaminergic, adenosinergic, melatoninergic, or orexinergic neurotransmission/ pathways to go beyond an influence on gaba-ergic neurotransmission. An already available new line of treatment consists in the application of orexin receptor agonists like suvorexant, which has just been recently admitted to the market in the US. This type of medication is based on orexin receptor antagonism and has been proven to produce clinically relevant effects on sleep in insomnia [ 178 , 179 ].
Potential for non-invasive brain stimulation approaches in the treatment of insomnia and depression
In addition to psycho-/pharmacotherapy and chronotherapeutic approaches, non-invasive brain stimulation techniques, including thermo-stimulation and transcranial direct current stimulation (tDCS), seem to have the potential to improve sleep. These techniques are able to induce local activity changes in specific cortical areas, which might modulate arousal and sleep via cortico-thalamo-cortical feedback loops. First proof-of-concept studies exist for the short-term induction of EEG slow waves by tDCS in healthy subjects [ 166 ] and a dose-dependent improvement of sleep latency and efficiency by frontal cerebral thermo-stimulation [ 180 ].
Another evolving strategy transforms recordings of brain activity patterns, such as EEG or real-time fMRI signals, into insomnia treatments by neurofeedback. These techniques aim at characterizing insomnia-related brain activity in order to allow patients to learn to control/ suppress specific patterns of brain activity with biofeedback [ 181 , 182 ]. Further work is necessary to evaluate whether neurofeedback can be helpful to treat insomnia and depression.
Circuit-based interrogation of a shared neurobiological pathogenesis of insomnia and depression using animal models
Rodent models of depression are widely used in drug discovery studies and a variety of tests has been developed to assess depressive behavior in rodents [ 183 , 184 ]. Surprisingly, sleep is rarely considered as outcome parameter in drug studies using animal models of depression. However, a cage exchange paradigm has been developed to investigate neural circuitry of stress-induced insomnia in rats inducing sleep disruption through mild stress and novelty of a depression [ 185 ]. This paradigm reproduces several of the polysomnographic features, which often accompany acute insomnia, namely sleep fragmentation, increased sleep onset latency, decreased NREM and REM sleep and an increase in high-frequency EEG activity during NREM sleep Interestingly, the regional assessment of neural activity using FOS expression, indicates a simultaneous activation of the sleep-promoting VLPO and arousal systems, leaving the sleep-wake regulatory switch in the brain in a hybrid state [ 185 ]. While these findings were acquired before the broad application of optogenetics to the neurobiological sleep research, the model provides a valuable basis for further delineation of neurophysiological mechanisms of insomnia in a rodent model
An intriguing opportunity to further disentangle the presumed hybrid state of sleep regulatory systems in insomnia arises from recent findings that many important aspects of sleep are regulated on a microscale [ 186 , 187 ]. Sleep homeostasis, the neuronal recovery process during NREM sleep, which is directly reflected by slow wave activity (0.5–4.0 Hz) during NREM, can strongly vary between individual regions depending on the previous use of cortical areas during wakefulness [ 188 , 189 , 190 ]. A hybrid pattern of signals characteristic for wakefulness and sleep can appear in the EEG of a wake and behaving animal after extensive training or prolonged wakefulness. Hybrid states of sleep microarchitecture have now also been reported in humans using intracerebral recordings acquired from patients undergoing diagnostic assessment for epilepsy [ 191 , 192 ]. Taken together, evidence from intracerebral recordings indicates a complex microarchitecture of vigilance states, which is not always observable on EEG recordings. Hybrid states on the microscale of either local cortical activation during sleep [ 191 ] or local cortical OFF states during wakefulness [ 190 , 192 ] can have remarkable behavioral consequences. These findings from basic research provide a putative explanation for the above-mentioned notion that subjective complaints of disrupted or non-restorative sleep are necessarily accompanied by polysomnographic findings (see section “What is insomnia?”). Further highly relevant basic research strategies encompass the modulation of neuroplasticity by sleep and the role of sleep as a key modulator of the neuro-immune axis.
Burton R. The anatomy of melancholy. NYRB Classic Imprint, Oxford; 1621.
Google Scholar
Strobl P. Die Macht des Schlafes in der griechisch-römischen Welt. Verlag Dr. Kovac: Hamburg; 2002.
Kraepelin E. Psychiatrie. JA Barth Publishing House (8th. edition); 1909.
Gustavsson A, Svensson M, Jacobi F, Allgulander C, Alonso J, Beghi E, et al. Cost of disorders of the brain in Europe 2010. Eur Neuropsychopharmacol. 2011;21:718–79.
CAS PubMed Google Scholar
Collins PY, Patel V, Joestl SS, March D, Insel TR, Daar AS. Grand challenges in global mental health. Nature. 2011;475:27–30.
CAS PubMed PubMed Central Google Scholar
Aserinsky E, Kleitman N. Regularly occuring periods of eye motility concomitant phenomena during sleep. Science. 1963;118:273–4.
Kupfer DJ, Foster FG. Interval between onset of sleep and rapid eye movement sleep as an indicator of depression. Lancet. 1972;2:648–9.
Kupfer DJ. REM latency: a psychobiological marker for primary depressive disease. Biol Psychiat. 1976;11:159–74.
Strawbridge R, Young AH, Cleare AJ. Biomarkers for depression: recent insights, current challenges and future prospects. Neuropsychiatr Dis Treat. 2017;13:1245–62.
Riemann D, Berger M, Voderholzer U. Sleep in depression: results from psychobiological studies. Biol Psychol. 2001;57:67–103.
Thase ME, Kupfer DJ, Spiker DG. Electroencephalographic sleep in secondary depression: a revisit. Biol Psychiat. 1984;19:805–14.
Benca RM, Obermeyer WH, Thisted RA, Gillin JC. Sleep and psychiatric disorders: a meta-analysis. Arch Gen Psychiat. 1992;49:651–68.
Baglioni C, Nanovska S, Regen W, Spiegelhalder K, Feige B, Nissen C, et al. Sleep and mental disorders: a meta-analysis of the last 20 years of polysomnographic research. Psychol Bull. 2016;142:969–90.
PubMed PubMed Central Google Scholar
Riemann D, Nissen C. Sleep and psychotropic drugs. In: Morin CM, Espie CA, editors. The Oxford Handbook of sleep and sleep disorders. Oxford: Oxford University Press; 2012.
Allocca G, Ma S, Martelli D, Cerri M, Del Vecchio F, Bastianini S, et al. Validation of ‘Somnivore’, a machine learning algorithm for automated scoring and analysis of polysomnography data. Front Neurosci. 2019. (In press).
Sitaram N, Nurnberger JI, Gershon ES, Gillin JC. Cholinergic regulation of mood and REM sleep: potential model and marker of vulnerability to affective disorder. Am J Psychiatry. 1982;139:571–6.
Hobson JA, McCarley RW, Wyzinski PW. Sleep cycle oscillation: reciprocal discharge by two brainstem neuronal groups. Science. 1975;189:55–8.
McCarley RW. REM sleep and depression: common neurobiological control mechanisms. Am J Psychiat. 1982;139:565–70.
Riemann D, Hohagen F, Bahro M, Lis S, Stadtmüller G, Gann H, et al. Cholinergic neurotransmission, REM sleep and depression. J Psychosom Res. 1994;38:15–25.
PubMed Google Scholar
Berger M, Riemann D, Höchli D, Spiegel R. The cholinergic rapid eye movement induction test with RS-86: state or trait marker of depression? Arch Gen Psychiatry. 1989;46:421–8.
Schreiber W, Lauer CJ, Krumrey K, Holsoboer F, Krieg JC, Cholinergic REM. induction test in subjects at high risk for psychiatric disorder. Biol Psychiatry. 1992;32:79–90.
Lauer CJ, Modell S, Schreiber W, Krieg JC, Holsboer F. Prediction of the development of a first major depressive disordere with a rapid eye movement sleep inducing. Test using the cholinergic agonist RS86. J Clin Psychopharmacol. 2004;24:356–7.
Wehr TA, Wirz-Justice A. Circadian rhythm mechanisms in affective ilnesss and in antidepressant drug action. Pharmacopsychiat. 1982;15:31–9.
CAS Google Scholar
Pflug B, Toelle R. Therapie endogener Depressionen durch Schlafentzug. Nervenarzt. 1971;42:117–24.
Borbely AA. A two process model of sleep regulation. Hum Neurobiol. 1982;1:195–204.
Borbely AA, Wirz-Justice A. Sleep deprivation and depression. A hypothesis derived from a model of sleep regulation. Hum Neurobiol. 1982;1:205–10.
Daan S, Beersma DGM, Borbély AA. Timing of human sleep: recovery process gated by a circadian pacemaker. Am J Physiol. 1984;246:R161–83.
Wehr TA, Wirz-Justice A, Goodwin FK. Phase advance of the circadian sleep-wake cycle as an antidepressant. Science. 1979;206:710–3.
Borbély AA, Daan S, Wirz-Justice A, Deboer T. The two-process model of sleep regulation: a reappraisal. J Sleep Res. 2016;25:131–43.
Germain A, Kupfer DJ. Circadian rhythm disturbances in depression. Hum Psychopharmacol. 2008;23:571–85.
Lewy AJ, Sack RL, Miller SL, Hoban TM. Antidepressant and circadian phase-shifting effects of light. Science. 1987;235:352–4.
Ehlers CL, Frank E, Kupfer DJ. Social zeitgebers and biological rhythms. A unified approach to undesrstanding the etiology of depression. Arch Gen Psychiatry. 1988;45:948–52.
Bunney BG, Bunney WE. Mechanisms of rapid antidepressant effects of sleep deprivation therapy: clock genes and circadian rhythms. Biol Psychiatry. 2013;73:1164–71.
Wu JC, Bunney WE. The biological basis of an antidepressant response to sleep deprivation and relapse: review and hypothesis. Am J Psychiat. 1990;147:14–21.
Dallaspezia S, Benedetti F. Chronobiology of bipolar disorder: therapeutic implication. Curr Psychiatry Rep. 2015;17:606.
Riemann D, Wiegand M, Lauer C, Berger M. Naps after total sleep deprivation in depressed patients: Are they depressiogenic? Psychiatry Res. 1993;49:109–20.
Landsness EC, Goldstein MR, Peterson MJ, Tononi J, Benca RM. Antidepressants effects of selective slow wave deprivation in major depression: a high-density EEG investigation. J Psychiat Res. 2011;45:1019–26.
Riemann D, Wiegand M, Berger M. Are there predictors for sleep deprivation response in depressed patients? Biol Psychiatry. 1991;29:707–10.
Svetska J. Sleep deprivation therapy. Neuro Endocrin Lett. 2008;29:65–92.
Castren E. Neuronal network plasticity and recovery from depression. JAMA Psychiatry. 2013;70:983–9.
Kuhn MA, Mainberger F, Feige B, Maier J, Mall V, Jung NH, et al. State-dependent partial occlusion of cortical LTP-like plasticity in major depression. Neuropsychopharmacology. 2016;41:1521–9.
Tononi G, Cirelli C. Sleep and the price of plasticity: from synaptic and cellular homeostasis to memory consolidation and integration. Neuron. 2014;81:12–34.
Kuhn M, Wolf E, Maier JG, Mainberger F, Feige B, Schmid H, et al. Sleep recalibrates homeostatic and associative synaptic plasticity in the human cortex. Nat. Commun. 2016;7:12455 https://doi.org/10.1038/ncomms12455.
Article CAS PubMed PubMed Central Google Scholar
Wolf E, Kuhn M, Normann C, Biber K, van Calker D, Riemann D, et al. Sleep and plasticity: potential mechanisms of therapeutic sleep deprivation in major depression. Sleep Med Rev. 2016;30:53–62.
Ngo HV, Martinetz T, Born J, Mölle M. Auditory closed loop stimulation of the sleep slow oscilation enhances memory. Neuron. 2013;78:545–53.
Fattinger S, de Beukelaar TT, Ruddy KL, Volk C, Heyse NC, Herbst JA, et al. Deep sleep maintains learning efficiency of the human brain. Nat Commun. 2017;8:15405.
Nofzinger EA, Buysse DJ, Germain A, Carter C, Luna B, Price JC, et al. Increased activation of anterior paralimbic and executive cortex from waking to rapid eye movement sleep in depression. Arch Gen Psychiatry. 2004;61:695–702.
Nofzinger EA, Buysse DJ, Germain A, et al. Alterations in regional cerebral glucose metabolism across waking and non-rapid eye movement sleep in depression. Arch Gen Psychiatry. 2005;62:387–96.
Nissen C, Nofzinger E. Sleep and depression: A functional neuroimaging perspective. In: Pandi-Perumal SR, Ruoti RR, Kramer M, editors. Sleep in psychosomatic medicine. Boca Raton: Taylor and Francis; 2007. p. 51–65.
American Psychiatric Association (APA). Diagnostic and statistical manual of mental disorders. 5th ed.: DSM-5. APA Publishing: Washington DC; 2013.
Riemann D, Spiegelhalder K, Feige B, Voderholzer U, Berger M, Perlis M, et al. The hyperarousal model of insomnia: A review of the concept and its evidence. Sleep Med Rev. 2010;14:19–31.
Morin C, Drake C, Harvey A, Krystal AD, Manber R, Riemann D et al. Insomnia disorder. Nature Reviews Disease Primers. 2015 epub first, https://doi.org/10.1038/nrdp.2015.26 .
Ohayon MM. Epidemiology of insomnia: What we know and what we still need to learn. Sleep Med Rev. 2002;6:97–111.
Qaseem A, Kansagara D, Forciea MA, Cooke M, Denberg TD. Management of chronic insomnia disorder in adults: A clinical practice guideline from the American College of Physicians. Ann Intern Med. 2016;165:889–97.
Riemann D, Baglioni C, Bassetti C, Bjorvatn B, Dolenc Groselj L, Hertenstein E, et al. European guideline for the diagnosis and treatment of insomnia. J Sleep Res. 2017;26:675–700.
Riemann D, Baum E, Cohrs S, Crönlein T, Hajak, G, Hertenstein E, et al. S3-Leitlinie Nicht erholsamer Schlaf/ Schlafstörungen–Kapitel “Insomnie bei Erwachsenen”, Update 2016. Somnologie. 2017;21:2–44. AWMF Leitlinie 063-003.
Riemann D, Spiegelhalder K, Nissen C, Hirscher V, Baglioni C, Feige B. REM sleep instability—a new pathway for insomnia? Pharmacopsychiatry. 2012;45:167–76.
Riemann D, Nissen C, Palagini L, Otte A, Perlis M, Spiegelhalder K. The neurobiology, investigation and treatment of chronic insomnia. Lancet Neurol. 2015;14:547–58.
Perlis M, Giles D, Mendelson W, Bootzin R, Wyatt J. Psychophysiological insomnia: The behavioural model and a neurocognitive perspective. J Sleep Res. 1997;6:179–88.
Saper CB, Scammell TE, Lu J. Hypothalamic regulation of sleep and circadian rhythms. Nature. 2005;437:1257–63.
Riemann D, Voderholzer U. Primary insomnia: a risk factor to develop depression? J Affect Disord. 2003;76:255–9.
Riemann D. Does effective management of sleep disorders reduce depressive symptoms and the risk of depression? Drugs. 2009;69:43–64.
Baglioni C, Battagliese G, Feige B, Nissen C, Voderholzer U, Lombardo C, et al. Insomnia as a predictor of depression: A meta-analytic evaluation of longitudinal epidemiological studies. J Affect Disord. 2011;135:10–9.
Li L, Wu C, Gan Y, Qu X, Lu Z. Insomnia and the risk of depression: a meta-analysis of prospective cohort studies. BMC Psychiat. 2016;16:375.
Bjørngaard JH, Bjerkeset O, Romundstad P, Gunnell D. Sleeping problems and suicide in 75,000 Norwegian adults: A 20 year follow-up of the HUNT I Study. Sleep. 2011;34:1155–9.
Pigeon WR, Pinquart M, Conner K. Meta-analysis of sleep disturbance and suicidal thoughts and behaviors. J Clin Psychiat. 2012;73:e1160–7.
Malik S, Kanwar A, Sim LA, Prokop LJ, Wang Z, Benkhadra K, et al. The association between sleep disturbances and suicidal behaviors in patients with psychiatric diagnoses: a systematic review and meta-analysis. Syst Rev. 2014;3:18.
Bernert RA, Turvey CL, Cornwell Y, Joiner TE. Association of poor subjective sleep quality with risk for death by suicide during a 10-year period. JAMA Psychiatry. 2014;71:1129–37.
Riemann D, Perlis ML. The treatments of chronic insomnia: a review of benzodiazepine receptor agonists and psychological and behavioral therapies. Sleep Med Rev. 2009;13:205–14.
Christensen H, Batterham PJ, Gosling JA, Ritterband LM, Griffiths KM, Thorndike FP, et al. Indicated prevention of major depressive disorder: A randomised controlled effectiveness trial of an online insomnia programme (SHUTi): The GoodNight study. Lancet Psychiatry. 2016;3:333–41.
Wirz-Justice A, Terman M, Oren DA, Goodwin FK, Kripke DF, Whybrow PC, et al. Brightening depression. Science. 2004;303:467–9.
Wirz-Justice A, Benedetti F, Terman M. Chronotherapeutics for affective disorders. 2nd ed. Basel: Karger; 2013.
Benedetti F, Barbini B, Colombo C, Smeraldi E. Chronotherapeutics in a psychiatric ward. Sleep Med Rev. 2007;11:509–22.
Baglioni C, Regen W, Tehgen A, Spiegelhalder K, Feige B, Nissen C, et al. Sleep changes in the disorder of insomnia: A meta-analysis of polysomnographic studies. Sleep Med Rev. 2014;18:195–213.
Wulff K, Gatti S, Wettstein JG, Foster RG. Sleep and circadian rhythm disruption in psychiatric and neurodegenerative disease. Nat Rev Neurosci. 2010;11:589–99.
Lu J, Sherman D, Devor M, Saper CB. A putative flip-flop switch for control of REM sleep. Nature. 2006;441:589–94.
Moruzzi G, Magoun HW. Brain stem reticular formation and activation of the EEG. Electro Clin Neurophysiol. 1949;1:455–73.
Li J, Hu Z, de Lecea L. The hypocretins/orexins: integrators of multiple physiological functions. Br J Pharmacol. 2014;171:332–50.
Chieffi S, Carotenuto M, Monda V, Valenzano A, Villano I, Precenzano F, et al. Orexin System: The key for a healthy life. Front Physiol. 2017;8:357.
Yeoh JW, Campbell EJ, James MH, Graham BA, Dayas CV. Orexin antagonists for neuropsychiatric disease: progress and potential pitfalls. Front Neurosci. 2014;8:36.
Liblau RS, Vassali A, Seifinejad A, Tafti M. Hypocretin (orexin) biology and the pathophysiology of narcolepsy with cataplexy. Lancet Neurol. 2015;14:318–28.
Diekelmann S, Born J. The memory function of sleep. Nat Rev Neurosci. 2010;11:114–26.
Maier JG, Kuhn M, Mainberger F, Guo S, Nachtsheim K, Bucsenec U, et al. Sleep orchestrates local plasticity and global stability of neural assemblies in the human cortex. Sleep. 2018;41:A43. (Abstract Supplement)
Kuhn M, Hertenstein E, Feige B, Landmann N, Spiegelhalder K, Baglioni C, et al. Declarative virtual water maze learning and emotional fear conditioning in primary insomnia. J Sleep Res. 2018;27:e12693 https://doi.org/10.1111/jsr.12693
Article PubMed Google Scholar
Van Calker D, Serchov T, Normann C, Biber K. recent insights into antidepressant therapy: distinct pathways and potential common mechanisms in the treatment of depressive syndromes. Neurosci Biobehav Rev. 2018;88:63–72.
Dunlap JC, Loros JJ, DeCoursey P. Chronobiology—biological timekeeping. Sunderland: Sinauer Association; 2004.
Akiskal HS, Bourgeois ML, Angst J, Post R, Möller H, Hirschfeld R. re-evaluating the prevalence of and diagnostic composition within the broad clinical spectrum of bipolar disorders. J Affect Dis. 2000; 59 (Suppl. 1): S5–S30.
Fernandes DC, Fogerson PM, Ospri LL, Thomsen MB, Layne RM, Severin D, et al. Light affects mood and learning through distinct retina-brain pathways. Cell. 2018;175:71–84.
Huang L, Peng Y, Yang Y, Huang X, Fu Y, Tao Q, et al. A visual circuit related to habenula underlies the antidepressive effects of light therapy. Neuron. 2019;102:128–42.
Zhou JN, Riemersma RF, Unmehopa UA, Hoogendijk WJ, van Heerikhuize JJ, Hofman MA, et al. Alterations in arginine vasopressin neurons in the suprachiasmatic nucleus in depression. Arch Gen Psychiatry. 2001;58:655–62.
Wu YH, Ursinus J, Zhou JN, Scheer FA, Ai-Min B, Jockers R, et al. Alterations of melatonin receptors MT1 and MT2 in the hypothalamic suprachiasmatic nucleus during depression. J Affect Disord. 2013;148:357–67.
Bernstein HG, Heinemann A, Krell D, Mawrin C, Bielau H, Danos P, et al. Further immunohistochemical evidence for impaired NO signaling in the hypothalamus of depressed patients. Ann N Y Acad Sci. 2002;973:91–3.
Plano SA, Golombek DA, Chiesa JJ. Circadian entrainment to light-dark cycles involves extracellular nitric oxide communication within the suprachiasmatic nuclei. Eur J Neurosci. 2010;31:876–82.
Dhir A, Kulkarni K. Nitric oxide and major depression. Nitric Oxide. 2011;30:125–31.
Kalsbeek A, Palm IF, La Fleur SE, Scheer FA, Perreau-Lenz S, Ruiter M, et al. SCN outputs and the hypothalamic balance of life. J Biol Rhythms. 2006;21:458–69.
Mendoza J. Circadian neurons in the lateral habenula: clocking motivated behaviors. Pharm Biochem Behav. 2017;162:55–61.
Sartorius A, Kiening KL, Kirsch P, von Gall CC, Haberkorn U, Unterberg AW, et al. Remission of major depression under deep brain stimulation of the lateral habenula in a therapy-refractory patient. Biol Psychiatry. 2010;67:e9–e11.
Clemm von Hohenberg C, Weber-Fahr W, Lebhardt P, Ravi N, Braun U, Gass N. Lateral habenula perturbation reduces default-mode network connectivity in a rat model of depression. Transl Psychiatry. 2018;8:68.
Gosnell SN, Curtis KN, Velasquez K, Fowler JC, Madan A, Goodman W, et al. Habnular connectivity may predict treatment response in depressed psychiatric inpatients. J Aff Dis. 2019;242:211–9.
Langel J, Ikeno T, Yan L, Nunez AA, Smale L. Distributions of GABAergic and glutamatergic neurons in the brains of a diurnal and nocturnal rodent. Brain Res. 2018;1700:152–9.
Lacoste B, Angeloni D, Dominguez-Lopez S, Calderoni S, Mauro A, Fraschini F, et al. Anatomical and cellular localization of melatonin MT1 and MT2 receptors in the adult rat brain. J Pineal Res. 2015;58:397–417.
Ciarleglio CM, Resuehr HE, McMahon DG. Interactions of the serotonin and circadian systems: nature and nurture in rhythms and blues. Neuroscience. 2011;197:8–16.
Spielman AJ, Caruso LS, Glovinsky PB. Behavioral perspective on insomnia. Psychiatr Clin North Am. 1987;10:541–53.
Palagini L, Biber K, Riemann D. The genetics of insomnia—evidence for epigenetic mechanisms. Sleep Med Rev. 2014;18:225–35.
Lahtinen A, Puttonen S, Vanttola P, Vitasalo K, Sulkava S, Perjakova N, et al. A distinctive DNA methylation pattern in insufficient sleep. Sci Rep. 2019;9:1193.
Hammerschlag AR, Stringer S, de Leeuw CA, Sniekers S, Taskesen E, Watanabe K, et al. Genome-wide associtaion analysis of insomnia complaints identifies risk genes and genetic overlap with psychtriac and metabolic traits. Nat Genet. 2017;49: 1584–92
Lane JM, Liang J, Vlasac I, Anderson SG, Bechtold DA, Bowden J, et al. Genom-wide association analyses of sleep disturbance traits identify new loci and highlight shared genetics with neuropsychiatric and metabolic traits. Nat Genet. 2017;49:274–81.
Lane JM, Jones S, Dashti HS, Wood AR, Aragam K, Van Hees V, et al. Biological and clinical insights from genetics of insomnia symptoms. Nat Genet. 2019;51:387–93.
Drake CL, Pillai V, Roth T. Stress and sleep reactivity: a prospective investigation of the stress-diathesis model of insomnia. Sleep. 2014;37:1295–304.
van de Laar M, Verbeek I, Pevernagie D, Aldenkamp A, Overeem S. The role of personality traits in insomnia. Sleep Med Rev. 2010;14:61–8.
Bastien CH, Vallières A, Morin CM. Precipitating factors of insomnia. Behav Sleep Med. 2004;2:50–62.
Healey E, Kales A, Monroe LJ, Bixler EO, Chamberlin K, Soldatos CB. Onset of insomnia: Role of life-stress events. Psychosom Med. 1981;43:439–51.
Feige B, Al-Shajlawi A, Nissen C, Voderholzer U, Hornyak M, Spiegelhalder K, et al. Does REM sleep contribute to subjective wake time in primary insomnia? A comparison of polysomnographic and subjective sleep in 100 patients. J Sleep Res. 2008;17:180–90.
Feige B, Nanovska S, Baglioni C, Bier B, Cabrera L, Diemers L, et al. Insomnia—perchance a dream? Results from a NonREM/ REM sleep awakening study in good sleepers and patients with insomnia. Sleep 2018;41: https://doi.org/10.1093/sleep/zsy032 .
Bootzin RR, Epstein D, Wood JM. Stimulus control instructions. In: Hauri P, editor. Case studies in insomnia. New York and London: Plenum;1991, p.19–28.
Carney CE, Harris AL, Moss TG, Edinger JD. Distinguishing rumination from worry in clinical insomnia. Behav Res Ther. 2010;48:540–6.
Baglioni C, Spiegelhalder K, Regen W, Feige B, Nissen C, Lombardo C, et al. Insomnia disorder is associated with increased amygdala reactivity to insomnia-related stimuli. Sleep. 2014;37:1907–17.
Baglioni C, Spiegelhalder K, Lombardo C, Riemann D. Sleep and emotions: a focus on insomnia. Sleep Med Rev. 2010;14:227–38.
Baglioni C, Lombardo C, Bux E, Hansen S, Salveta C, Biello S, et al. Psychophysiological reactivity to sleep-related emotional stimuli in primary insomnia. Behav Res Ther. 2010b;48:467–75.
LeDoux J. The amygdala. Curr Biol. 2007;17:868–74.
Sakurai T, Mieda M. Connectomics of orexin-producing neurons: Interface of systems of emotion, energy homeostasis and arousal. Trends Pharm Sci. 2011;32:451–62.
Ballesta A, Innominato PF, Dallmann R, Rand DA, Lévi FA. Systems chronotherapeutics. Pharm Rev. 2017;69:161–99.
Sateia MJ, Buyyse DJ, Krystal AD, Neubauer DN, Heald JL. Clinical practice guidelines for the pharmacologic treatment of chronic insomnia in adults: an American academy of sleep medicine clinical practice guideline. J Clin Sleep Med. 2017;13:307–49.
Perlis ML, Jungquist C, Smith MT, Posner D. Cognitive behavioural treatment of insomnia - A session-by-session guide. Springer: New York; 2005.
Harvey AG. A cognitive model of insomnia. Behav Res Ther. 2002;40:869–93.
Espie CA, Broomfield NM, MacMahon KM, Macphee LM, Taylor LM. The attention-inattention-effort pathway in the development of psychophysiologic insomnia: an invited theoretical review. Sleep Med Rev. 2006;10:215–45.
Miller CB, Espie CA, Epstein DR, Friedmann L, Morin CM, Pigeon WR, et al. The evidence base of sleep restriction therapy for treating insomnia disorder. Sleep Med Rev. 2014;18:415–24.
Maurer LF, Espie CA, Kyle SD. How does sleep restriction therapy for insomnia work? A systematic review of mechanistic evidence and the introduction of the Triple-R model. Sleep Med Rev. 2018;42:127–38.
Mendelson WB, Roth T, Cassella J, Roehrs T, Walsh JK, Woods JH, et al. The treatment of chronic insomnia: drug indications, chronic use and abuse liability. Summary of a 2001 new clinical drug evaluation unit meeting symposium. Sleep Med Rev. 2004;8:7–17
Barker MJ, Greenwood KM, Jackson M, Crowe SF. Persistence of cognitive effects after withdrawal from longterm benzodiazepine use: ameta-analysis. Arch Clin Neuropsychol. 2004;19:437–54.
Dassanayake T, Micie P, Carter G, Jones A. Effects of benzodiazepines, antidepressants, and opoids on driving. A systematic review and meta-analysis of epidemiological and experimental evidence. Drug Saf. 2011;34:125–56.
Hajak G, Müller WE, Wittchen HU, Pittrow D, Kirch W. Abuse and dependence potential for the non-benzodiazepine hypnotics Zolpidem and Zopiclone - A review of case reports and epidemiological data. Addiction. 2003;98:1371–78.
Ashton H. The diagnosis and management of benzodiazepine dependence. Curr Opin Psychiatry. 2005;18:249–55.
MacFarlane J, Morin CM, Montplaisir J. Hypnotics in insomnia: the experience of zolpidem. Clin Ther. 2014;36:1676–701.
Kripke DF, Garfinkel I, Wingard DL. Mortality associated with sleep duration and insomnia. Arch Gen Psychiatry. 2002;59:131–6.
Kripke DF. Do hypnotics cause death and cancer? The burden of proof. Sleep Med. 2009;10:275–6.
Neutel CL, Johansen HL. Association between hypnotic use and increased mortality: causation or confounding? Eur J Pharm. 2015;71:637–42.
Pottegard A, Friis S, Andersen M, Hallas J. Use of benzodiazepines or benzodiazepine related drugs and the risk of cancer: a population-based case-control study. Br J Clin Pharm. 2013;75:1356–64.
McGechan A, Wellington K. Ramelteon. CNS Drugs. 2005;19:1057–65.
Herxheimer A, Petrie KJ. Melatonin for the prevention and treatment of jet lag. Issue 4. Oxford: The Cochrane Library; 2002
Buscemi N, Vandermeer B, Hooton N, Pandiaya R, Tjosvold L, Hartling L, et al. The efficacy and safety of exogenous melatonin for primary sleep disorders. J Gen Int Med. 2005;20:1151–8.
Buscemi N, Vandermeer B, Hooton N, Pandiaya R, Tjosvold L, Hartling L, et al. Efficacy and safety of exogenous melatonin for secondary sleep disorders accompanying sleep restriction: meta-analysis. Brit Med J. 2006;332:1–9. (published online 10 February)
Sateia MJ, Kirby-Long P, taylor JL. Efficacy and clinical safety of ramelteon: an evidence-based review. Sleep Med Rev. 2008;12:319–32.
Frase L, Nissen C, Riemann D, Spiegelhalder K. Making sleep easier: pharnacological interventions for insomnia. Expert Opin Pharmacother. 2018;19:1465–1473.
Kuriyama A, Honda M, Hayashino Y. Ramelteon for the treatment of insomnia in adults: a systematic review and meta-analysis. Sleep Med Rev. 2014;15:385–92.
Davis KL, Charney D, Coyle JT, Nemeroff C. Neuropsychopharmacology. Philadelphia: Lippincott, Williams & Wilkins; 2002.
Walsh JK, Schweitzer PK. Ten-year trends in the pharmacological treatment of insomnia. Sleep. 1999;22:371–5.
Walsh JK. Drugs used to treat insomnia in 2002: regulatory-based rather than evidence-based medicine. Sleep. 2004;27:1441.
Walsh JK, Erman M, Erwin M, Jamieson A, Mahowald M, Regestein Q, et al. Subjective hypnotic efficacy of trazodone and zolpidem in DMS-III-R primary insomnia. Hum Psychopharmacol. 1998;13:191–8.
Hajak G, Rodenbeck A, Voderholzer U, Riemann D, Cohrs S, Hohagen F, et al. Doxepin in the treatment of primary insomnia: A placebo-controlled, double-blind, polysomnographic study. J Clin Psychiat. 2001;62:453–63.
Riemann D, Voderholzer U, Cohrs S, Rodenbeck A, Hajak G, Rüther E, et al. Trimipramine in primary insomnia: results of a polysomnographic double-blind controlled study. Pharmacopsychiatry. 2002;35:165–4.
Winkler A, Auer C, Doering BK, Rief W. Drug treatment of primary insomnia: a meta-analysis of polysomnographic radomized controlled trials. CNS Drugs. 2014;28:799–816.
Roth T, Rogowski R, Hull S, Schwartz H, Koshorek G, Corsev B, et al. Efficacy and safety of doxepin 1 mg, 3 mg, and 6 mg in adults with primary insomnia. Sleep. 2007;30:1555–61.
Cohrs S. Sleep disturbances in patients with schizophrenia. CNS Drugs. 2008;22:939–62.
McCall C, Vaughn McCall W. What is the role of sedating antidepressants, antipsychotics and antoconvulsants in the management of insomnia? Curr Psychiatry Rep. 2012;14:494–502.
Anderson SL, Vande Griend JV. Quetiapine for insomnia: a review of the literature. Am J Health-Syst. 2014;71:394–402.
Barbini B, Benedetti F, Colombo C, Dotoli D, Bernasconi A, Cigala-Fulgosi M, et al. Dark therapy for mania: a pilot study. Bipolar Disord. 2005;7:98–101.
Frank E, Swartz HA, Kupfer DJ. Interpersonal and social rhythm therapy: managing the chaos of bipolar diorder. Biol Psychiat. 2000;48:593–604.
Frank E, Kupfer DJ, Thase M, Mallinger AG, Swartz HA, Fagioloni AM, et al. Two-year outcomes for interpersonal and social rhythm therapy in individuals with bipolar I disorder. Arch Gen Psychiat. 2005;62:996–1004.
Van Geilswijk IM, Korzilius HP, Smits MG. The use of exogenous melatonin in delayed sleep phase disorder: a meta-analysis. Sleep. 2010;33:1605–14.
Liira J, Verbeek JH, Costa G, Driscoll TR, Sallinen M, Isotalo LK, et al. Pharmacological interventions for sleepiness and sleep disturbances caused by shift work. Cochr Database Syst Rev. 2014; CD009776.
Spadoni G, Bedini A, Rivara S, Mor S. Melatonin receptor agonists: new options for insomnia and depression treatment. CNS Neurosci Ther. 2011;17:733–41.
Phillips L, Appleton RE. Systematic review of melatonin treatment in children with neurodevelopmental disabilities and sleep impairment. Dev Med Child Neurol. 2004;45:771–5.
Krone L, Frase L, Piosczyk H, Kuhn M, Selhausen P, Zittel S, et al. Top-down control of arousal and sleep and potential clinical implications. Sleep Med Rev. 2017;31:17–24.
Nitsche MA, Paulus W. Excitability changes induced in the human motor cortex by weak transcranial direct current stimulation. J Physiol. 2000;527:633–9.
Marshall L, Helgadóttir H, Mölle M, Born J. Boosting slow oscillations during sleep potentiates memory. Nature. 2006;444:610–3.
Frase L, Piosczyk H, Zittel S, Jahn F, Selhausen P, Krone L, et al. The modulation of arousal and sleep continuity by transcranial direct current stimulation (tDCS). Neuropsychopharmacology. 2016;41:2577–86.
Frase L, Selhausen P, Krone L, Zittel S, Jahn F, Feige B, et al. Transcranial direct current stimulation (tDCS) in insomnia disorder. Brain Stimulation. 2019 (in press).
Espie CA. “Stepped care”: a health technology solution for delivering cognitive behavioral therapy as a first line insomnia treatment. Sleep. 2009;32:1549–58.
Espie CA, Kyle S, Williams C, Ong J, Douglas NJ, Hames P, et al. A randomized, placebo-controlled trial of online cognitive-behaviroal therapy for chronic insomnia disorder delivered via an automated media-rich web application. Sleep. 2012;35:769–81.
Espie CA, MacMahon KMA, Kelly H-L, Broomfield NM, Douglas NJ, Engleman HM, et al. Randomized clinical effectiveness trial of nurse-administered small-group cognitive behavior therapy for persistent insomnia in general practice. Sleep. 2007;30:574–84.
Buysse DJ, Germain A, Moul DE, Franzen PL, Brar LK, Fletcher ME, et al. Efficacy of brief behavioral treatment for chronic insomnia in older adults. Arch Intern Med. 2011;171:887–95.
Ellis J, Cushing T, Germain A. Treating acute insomnia: a randomized controlled trioal of a “single-shot” of cognitive behavioral therapy for insomnia. Sleep. 2015;38:971–8.
Espie CA, Emsley R, Kyle SD, Gordon C, Drake CL, Siriwardena AN, et al. Effect of digital cognitive behavioral therapy for insomnia on health, psychological well-being, and sleep-related quality of life: a randomized clinical trial. JAMA Psychiatry. 2019;19:21–30.
Geiger-Brown JM, Rogers VE, Liu W, Ludeman EM, Downton KD, Diaz-Abad M. Cognitive behavioral therapy in persons with comorbid insomnia: a meta-analysis. Sleep Med Rev. 2015;23:54–67.
Wu JQ, Appleman ER, Salazar RD, Ong J. Cognitive behavioral therapy for insomnia comorbid with psychiatric and medical conditions: a meta-analysis. JAMA Intern Med. 2015;175:1461–72.
Gosling JA, Glozier N, Griffiths K, Ritterband L, Thorndike F, Mackinnon A, et al. The GoodNight study—online CBT for insomnia for the indicated prevention of depression: study protocol for a randomised controlled trial. Trials. 2014;15:56.
Michelson D, Snyder E, Paradis E, Chengau-Lin M, Snavely DB, Hutzelman J, et al. Safety and efficacy of suvorexant during 1-year treatment of insomnia with subsequent abrupt treatment discontinuation: a phase 3 randomised, double-blind, placebo-controlled trial. Lancet Neurol. 2014;13:461–71.
Riemann D, Spiegelhalder K. Orexin receptor antagonists: a new treatment for insomnia? Lancet Neurol. 2014;13:441–3.
Nofzinger E, Buysse DJ. Frontal cerebral thermal transfer as a treatment for insomnia: a dose-ranging study. Sleep. 2011;34:A0534.
Sulzer J, Haller S, Scharnowski F, Weiskopf N, Birbaumer N, Biefasi ML, et al. Real-time fMRI neurofeedback: progress and challenges. Neuroimage. 2013;76:386–99.
Schabus M, Heib DP, Lechinger J, Griessenberger H, Klimesch W, Pawlizki A, et al. Enhancing sleep quality and memory in insomnia using instrumental sensorimotor rhythm conditioning. Biol Psychol. 2014;95:126–34.
O’Leary OF, Cryan JF. Towards translational rodent models of depression. Cell Tissue Res. 2013;354:141–53.
Ramaker MJ, Dulawa SC. Identifying fast-onset antidepressants using rodent models. Mol Psychiatry. 2017;22:656.
Cano G, Mochizuki T, Saper CB. Neural circuitry of stress-induced insomnia in rats. J Neurosci. 2008;28:10167–84.
Krueger JM, Rector DM, Roy S, Van Dongen HPA, Belenky G, Panksepp J. Sleep as a fundamental property of neuronal assemblies. Nat Rev Neurosci. 2008;9:910–9.
Krueger JM, Tononi G. Local use-dependent sleep; synthesis of the new paradigm. Curr Top Med Chem. 2011;11:2490–2.
Kattler H, Dijk D-J, Borbély AA. Effect of unilateral somatosensory stimulation prior to sleep on the sleep EEG in humans. J Sleep Res. 1994;3:159–64.
Huber R, Felice Ghilardi M, Massimini M, Tononi G. Local sleep and learning. Nature. 2004;430:78–81.
Vyazovskiy VV, Olcese U, Hanlon EC, Nir Y, Cirelli C, Tononi G. Local sleep in awake rats. Nature. 2011;472:443–7.
Nobili L, Ferrara M, Moroni F, De Gennaro L, Russo GLo, Campus C, et al. Dissociated wake-like and sleep-like electro-cortical activity during sleep. Neuroimage. 2011;58:612–9.
Nir Y, Andrillon T, Marmelshtein A, Suthana N, Cirelli C, Tononi G, et al. Selective neuronal lapses precede human cognitive lapses following sleep deprivation. Nat Med. 2017;23:1474–80.
Saper CB, Fuller PM, Pedersen NP, Lu J, Scammell TE. Sleep state switching. Neuron. 2010;68:1023–42.
Download references
Funding and disclosure
None of the authors declares any competing financial interest in relation to the work described. Concerning the pharmaceutical industry, DR received honoraria from HEEL pharmaceuticals in 2017/2018 for one congress presentation each. DR is a member of the executive board of Freiburg Institute for Behavioral Therapy and thus receives honoraria for regular meetings, examinations, and presentations in this context. DR frequently lectures at hospitals, universities, and other institutions of education about sleep, insomnia, and mental health—frequently, he receives a honorarium from these institutions for his work. DR receives royalties from several publishing houses for his books on sleep and insomnia. DR receives payment from the European Sleep Research Society for his duty as Editor in Chief of the Journal of Sleep Research. The other authors declare no competing interests.
Author information
Authors and affiliations.
Department of Psychiatry and Psychotherapy, Medical Center—University of Freiburg, Faculty of Medicine, University of Freiburg, Freiburg, Germany
Dieter Riemann
Sleep and Circadian Neuroscience Institute, University of Oxford, Oxford, UK
Lukas B. Krone
Department of Physiology, Anatomy, and Genetics, University of Oxford, Oxford, UK
Departments of Radiation Sciences & Molecular Biology, Umea University, Umeå, Sweden
Katharina Wulff
Wallenberg Centre for Molecular Medicine (WCMM), Umea University, Umeå, Sweden
University Hospital of Psychiatry and Psychotherapy, Bern, Switzerland
Christoph Nissen
You can also search for this author in PubMed Google Scholar
Corresponding author
Correspondence to Dieter Riemann .
Additional information
Publisher’s note: Springer Nature remains neutral with regard to jurisdictional claims in published maps and institutional affiliations.
Rights and permissions
Reprints and permissions
About this article
Cite this article.
Riemann, D., Krone, L.B., Wulff, K. et al. Sleep, insomnia, and depression. Neuropsychopharmacol. 45 , 74–89 (2020). https://doi.org/10.1038/s41386-019-0411-y
Download citation
Received : 02 March 2019
Revised : 21 April 2019
Accepted : 23 April 2019
Published : 09 May 2019
Issue Date : January 2020
DOI : https://doi.org/10.1038/s41386-019-0411-y
Share this article
Anyone you share the following link with will be able to read this content:
Sorry, a shareable link is not currently available for this article.
Provided by the Springer Nature SharedIt content-sharing initiative
This article is cited by
Association between insomnia and depressive symptoms among law enforcement personnel: the moderating role of resilience.
- Danya M. Serrano
- Katrina A. Rufino
- Anka A. Vujanovic
Journal of Police and Criminal Psychology (2024)
Überblick zu chronobiologischen und schlafmedizinischen Aspekten bei Depressionen im Jugendalter
- Neda Ghotbi
- Aline Doreen Scherff
- Gerd Schulte-Körne
Bundesgesundheitsblatt - Gesundheitsforschung - Gesundheitsschutz (2024)
Sleep is Essential for Mental Health: Potential Role of Slow Oscillations
- Giulia Aquino
- Gaspare Alfì
- Angelo Gemignani
Current Sleep Medicine Reports (2024)
The assessment of fatigue and sleep quality among children and adolescents with familial Mediterranean fever: A case-control and correlation study
- Çağla İncesu
- Gülşah Kavrul Kayaalp
- Rukiye Nurten Ömeroğlu
European Journal of Pediatrics (2024)
Sleep behaviors predicted sleep disturbances among Chinese health science students: a cross-sectional study
- Jinjin Yuan
- Bingqian Zhu
Sleep and Breathing (2024)
Quick links
- Explore articles by subject
- Guide to authors
- Editorial policies

Sleep Deprivation Is Slowing Your Metabolism. Here's What to Do About It
You're not dreaming -- sleep loss can affect your weight. These tips can help.

If you're reaching for snacks more than usual and you can't quite work up the energy to go for a walk, a slowing metabolism could be at fault. And if you're tossing and turning at night? Well, those two things could be related.
March is Sleep Awareness Month, but it's always a good time to consider whether you're getting enough rest. Lack of sleep can not only make you feel groggy and slow down your reaction times but can also affect your body on a cellular level. If you experience chronic sleep loss, you may experience a slower metabolism and increased hunger. Luckily, there are things you can do about it.
Read more : Can Tech Help You Sleep Better? This Is My 3-Week Quest for Answers
The connection between sleep and metabolism

While losing a few hours of sleep once a week isn't likely to affect your long-term metabolic rate, some research suggests that sleep and metabolism are interconnected. Losing sleep can affect your glucose metabolism, which affects how your body breaks down sugars and also changes the way your body metabolizes fats.
What is metabolism?
As Harvard Health Publishing explains, metabolism refers to the process by which your body expends energy and burns calories around the clock. This essential operation helps your body do everything from breaking down food to breathing and repairing cells. While your metabolic rate is largely determined by genes, natural processes like aging can affect it, as well as variables such as sleep and level of activity.
Does sleeping speed up metabolism?
When you're asleep, your metabolism slows by about 15% . Conversely, if you don't get enough sleep, your waking metabolism may slow. For most adults, the sweet spot is between 7 and 9 hours of sleep nightly to get adequate rest and keep your metabolism at its baseline.
In addition to getting enough sleep, working out and eating well may give your metabolism a boost. Keep in mind that it's common for metabolism rates to decline with age.
Effects of sleep deprivation on your metabolism
Multiple systems in your body may be impacted by sleep deprivation. Research conducted at Penn State found that restricting sleep for 10 days could begin to affect the way your body metabolizes fats. Another study published in the Science Advances journal found that acute sleep loss results in genome-wide DNA changes, including metabolite levels. The National Library of Medicine says that losing sleep may alter your glucose metabolism and other hormones in your body that regulate how quickly you burn energy.
Other effects of sleep deprivation may include:
- Appetite disregulation
- Lower energy expenditure
- Insulin resistance
- Inability to concentrate
- Impaired memory
Can too much sleep cause weight gain?
Since our metabolic rate slows when we sleep, and we're not burning a lot of calories while we slumber , it is possible for too much sleep to cause weight gain. Increased activity helps you burn calories and may allow you to maintain a better baseline metabolism.
5 tips for improving sleep quality
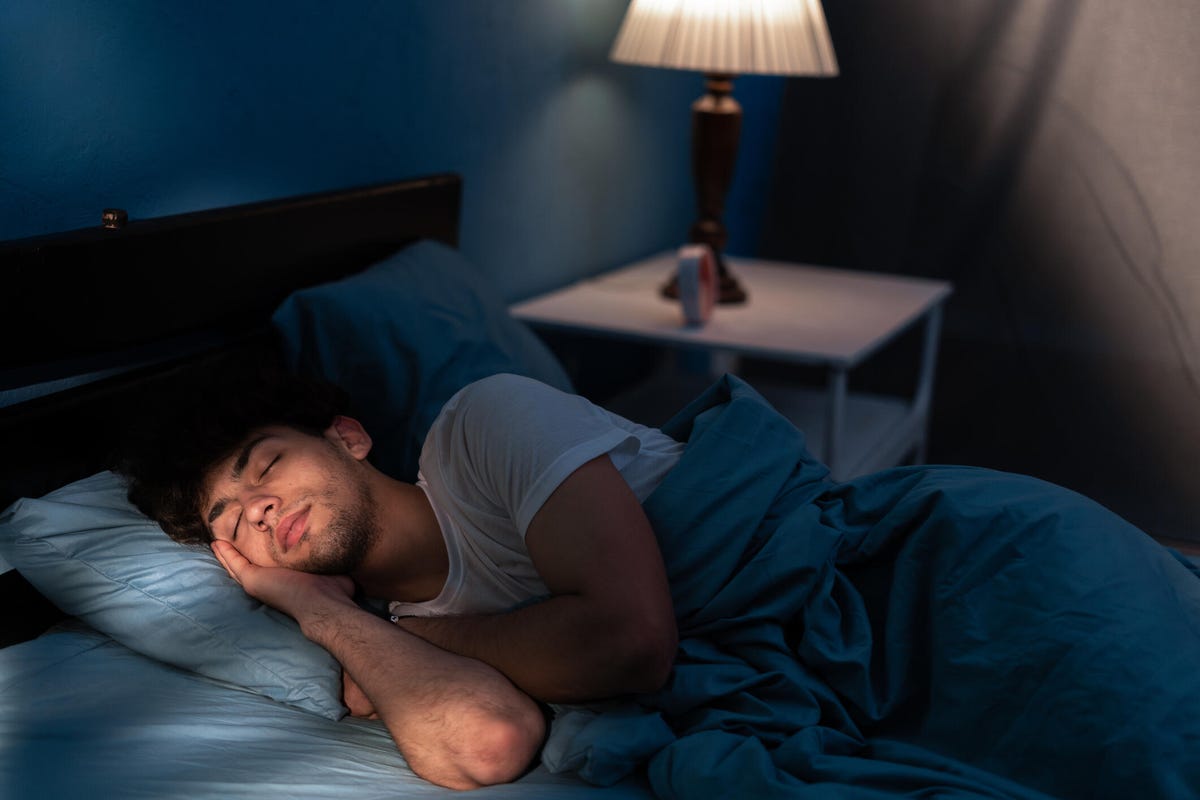
If you're struggling with good sleep, making lifestyle changes is a good place to start. The following tips may be enough to encourage more rest and avoid negative changes to your metabolism.
Wake up and go to bed at the same time
Sleep consistency may help you get better sleep because it encourages a more reliable circadian rhythm . This process is a natural body clock that runs on a 24-hour cycle and tells you when to be alert and when to get sleepy. Irregular sleep times may disrupt your rhythm and confuse your body about when it's supposed to get tired.

Block out light
Darkness can also encourage your circadian rhythm to stay regular. Light sends the signal to your brain that it's time to be alert, while darkness tells it to rest. Try a sleep mask or blackout curtains in your bedroom if you're having a hard time getting to sleep. Looking at smartphones and other screens right before bed may also prohibit good sleep, so put them away an hour before you plan to sleep.
Try natural sleep aids
You may also benefit from natural sleep aids that can be purchased over the counter. This includes melatonin, which is a compound that naturally occurs in the brain when it's time to sleep but can be supplemented in pill form. You may also want to try drinking herbal tea or using an essential oil diffuser to get relaxed before bedtime.
Maintain good sleep hygiene
Keeping your bed sheets , pillows, and blankets clean may also promote good sleep. Make sure your bedding is comfortable, and your mattress is supportive, and make sure your nightstand is in order. These small changes can encourage your body to relax when you lie down to sleep.
Avoid caffeine and alcohol before bed
Caffeine, alcohol and even big meals can mess with your ability to fall asleep quickly. Try to eliminate caffeine from your diet later in the day and limit food and alcohol for a few hours before bed. Spicy foods, even in small amounts, may also deprive you of good sleep by causing heartburn. Other foods like whole grains, fruits and nuts may be better foods to eat for sleep .
When to seek a doctor
Not all sleep issues can be remedied with lifestyle changes. There are times when it could benefit you to seek a doctor's opinion. Keep the following in mind:
Getting more sleep may not be a good weight loss plan. Often, the most effective way to lose weight is highly personalized and includes more than improving your sleep schedule. If you are struggling with weight loss, consider contacting a dietician or medical doctor.
Sleep deprivation might be caused by a sleeping disorder. Do you struggle to sleep once in a while, or is the problem more chronic, lasting for weeks or months? If your loss of sleep is a long-term problem, it's wise to get a medical opinion.
Negative side effects may need to be treated professionally. If you're not getting enough sleep, you could start to experience chronic fatigue, lack of concentration, or other symptoms that affect your everyday life. If you find that your regular sleep deprivation is affecting your ability to function day-to-day, talk to a doctor.
Mattress Buying Guides
- Best Mattress
- Best Air Mattress
- Best Adjustable Mattress
- Best Mattress in a Box
- Best Memory Foam Mattress
- Best Mattress for Side Sleepers
- Best Mattress for Stomach Sleepers
- Best Mattress for Back Pain
- Best Mattress for Heavy People
- Best Mattress for Kids
- Best Cooling Mattress
- Best Cheap Mattress
- Best Firm Mattress
- Best Soft Mattress
- Best King Mattress
- Purple Mattress
- Dreamcloud Mattress
- Nectar Mattress
- Casper Mattress
- TempurPedic Mattress
- Saatva Mattress
- Tuft & Needle Mattress
- Helix Mattress
- Avocado Mattress
Other Sleep Guides
- Best Pillow
- Best Weighted Blanket
- Best Sleep Mask
- Best Sheets
- Best Mattress Toppers
- Best Mattress Pads
- Best Headphones for Sleeping
- Best Alarm Clock
- Best Earplugs for Sleeping
- Best White Noise Machines
- Best Products for Snoring
- Skip to main content
- Keyboard shortcuts for audio player

Your Health
- Treatments & Tests
- Health Inc.
- Public Health
A new podcast examines the perils of intense meditation
Andrea Muraskin

Imagine it's a crisp clear winter day, and you're skiing down a mountain, feeling exhilarated. All of a sudden, you lose control of your skis. You're hurtling down towards the base of the slope, and all you can feel is abject terror.
That's how one young man explained his emotional state during an intensive meditation retreat. It was one of several troubling accounts reporter Madison Marriage heard while reporting Untold: The Retreat , a new investigative podcast series from the Financial Times and Goat Rodeo.
The four-episode series focuses on retreats held by the Goenka network, teaching a popular meditation technique called Vipassana. Participants follow a strict schedule, waking before dawn and meditating silently for 10 days, 10 hours per day. They eat just two vegan meals each day.

In Oregon, psilocybin treatment is an experiment in real time
Meditation and mindfulness have many known health benefits, including helping to process trauma and manage anxiety , improve eating habits, and ease chronic pain. While many participants say Goenka retreats changed their lives for the better, The Retreat tells the stories of individuals whose mental health deteriorated during a 10 day retreat – or for some, after several 10-day retreats.
Some spent time in psychiatric units, and two participants whose families spoke to Marriage, took their own lives.
If you or someone you know may be considering suicide or is in crisis, call or text 988 to reach the Suicide & Crisis Lifeline .
Marriage interviewed nearly two dozen people who had attended Goenka retreats in different countries, including the U.K., the United States, France, India, and Australia. According to these former participants, retreat staff all over the world had a similar reaction when they were approached with mental health problems. "They're going to be telling you the same thing, which is keep meditating even if you're in severe emotional distress," she told NPR.
A global organization, the structure of the Goenka network is decentralized. The Financial Times reached out for comment to lead teachers at several Goenka centers, including the centers in Delaware and British Columbia where participants had died by suicide after exhibiting signs of psychological distress. But they declined to do an interview or answer specific questions on the record.

Author Interviews
In 'hidden valley road,' a family's journey helps shift the science of mental illness.
Bob Jeffs, director of one Goenka center near Merritt, British Columbia, told the producers of The Retreat in a written statement that his staff assess applicants before retreats and tries to dissuade people who are not ready: "Although the experience of hundreds of thousands of people who have successfully completed retreats since the early 1970's is overwhelmingly positive, these courses are not for everyone. We take the safety and well-being of every student in our care extremely seriously."

Untold: The Retreat is a podcast from The Financial Times and Goat Rodeo. The Financial Times hide caption
NPR contributor Andrea Muraskin spoke with Marriage about what her investigation uncovered about the mental health risks of meditation retreats.
This interview has been edited for length and clarity.
Andrea Muraskin: What is Vipassana meditation and how is it taught at Goenka retreats?
Madison Marriage: Vipassana meditation is a type of meditation, which is ancient, its roots go back thousands of years... These retreats teach Vipassana meditation through the teachings of S. N. Goenka. And he's a kind of guru at the heart of this network, who founded the first meditation retreats back in the 1970s, and they've really proliferated.
Goenka's technique is that you spend a few days focusing on just one area of your body, and then it expands. And you have to shift your focus to different parts of your body. You wake up at 4 a.m., you start meditating at 4:30 a.m. You have a break at specific times, your day ends at 8, 9 p.m. And then in theory, you go to bed.
Muraskin: What did you discover about the Goenka retreats and mental health?
Mariage: I don't think many people associate the word meditation with anything negative. It sounds relaxing and something that you might do to help soothe yourself. And that's exactly the reason why a lot of people go off and do these retreats. They're looking for something that's going to help them to feel a bit more relaxed, a bit more calm, having a better headspace, that kind of thing.
I've now interviewed dozens of people who've done these retreats and have had the complete adverse reaction. It's almost like kind of jumping off a cliff in terms of their mental health. Some of these people have done two retreats or three retreats or ten retreats and really loved them. But there is a specific retreat where something in their mind clicks or breaks or snaps. Those are the kind of words that they've used.
Psychosis is really common. So [are] hallucinations, physical pain, like electrical zaps going up and down their bodies. In the first episode, [one young woman] describes it as being like stuck in a torture chamber for her mind.
The big one is terror, abject terror. I had one person email me this week saying, 'Thank you for making this podcast because I thought I was alone.' And he said that he would rather saw his own arm off than go back to that mental headspace.
One man in Britain ...was escorted out of a Goenka center in handcuffs by the police because he had to be sectioned at the local hospital and he wouldn't go voluntarily. There are people leaving these centers and heading to psychiatric units.
Muraskin: What did you learn about what's happening in the brains of people who have these adverse experiences with meditation?
Mariage: So we've interviewed several experts about what meditation does to the brain and one of the foremost experts we spoke to said it's a bit like a stimulant. So having lots of coffee or too much of any stimulants can end up having the opposite effect where instead of doing something good for you, it starts doing something bad, and it can begin to feel a little bit addictive. But there are limits to what the scientific community knows about the human brain and how and why it works in certain ways.
Muraskin: One of your interviewees told you she felt as if she had become addicted to meditation. There's no official diagnosis for meditation addiction in psychology. But did you speak to others who had experiences similar to addiction?
Mariage: Yes. Lots of people said that their first retreat or first several retreats really helped them and really brought them to quite an exciting spiritual plane. It almost sounds kind of mystical and godlike – you're on cloud nine mentally, and they come out and they feel calmer. They know how to process their thoughts better. Their life feels easier as a result. So they go to another. And they have kind of similar feelings, maybe not quite as intense.
And then the feeling starts to fade. So they do another retreat. And then a lot of people said that they ended up struggling to sleep. So they would meditate more because they had initially felt that meditation would help them to sleep because it had made them feel calmer at first. But effectively, they end up meditating through the night, all day, every day for weeks or months on end.
And then, I think maybe this comes back to your earlier question about impact on the brain – I would argue it's perhaps not meditation per se that is harming people's brains. A lot of the people I spoke to ended up having severe sleep deprivation. And it is clinically proven to be extremely bad for your brain not to sleep.
Muraskin: We've heard from several of our readers over time that they benefit from mindfulness and meditation. If somebody reading this interview becomes concerned, and thinks, I like my meditation practice, but should I be worried now, what would you say to someone like that?
Mariage: So the consensus from the psychologists and psychiatrists and academics I spoke to is that amounts of meditation up to half an hour a day on the whole is usually completely fine.
[The problem is] the extremity of this particular practice. Ten hours a day of meditating without any physical movement. You're sitting on the floor cross-legged with your eyes closed, meditating for 10 hours a day. You're put on a vegan diet. So for a lot of people that's far fewer calories, often at half of what they're usually used to. And there's no dinner. There's an element of sleep deprivation. And your sensory world is being massively diminished. And it's that which I think is driving people to quite extreme outcomes.
Muraskin: Do you think the psychological problems that came up during retreats could be explained by underlying mental health issues that the meditators had before they began meditating?
Mariage: I think that's a really difficult question because how can anyone know whether they have a mental health problem? You're meant to fill out a form before you go to one of these retreats and state whether or not you've ever had any kind of mental health issue or history of drug abuse. And if you've never had a mental health problem, you will of course say no and no, and in you go.
And I've spoken to people who say that they were completely stable prior to doing one of these retreats, had never had a mental or physical problem in their lives, and had never tried drugs, and they have gone in and they have emerged completely broken.
I actually think it's irrelevant whether or not somebody had a mental health issue beforehand, because the evidence that I've seen is that the particular format of these retreats can push people past their limits.
Muraskin: Based on your interviews with participants, is it difficult to leave a Goenka retreat early?
Mariage: Yes, it is difficult to leave a retreat early. [If you express the desire to], you're effectively gaslighted into staying.
You're told, oh, you might just be on the cusp of a breakthrough. The founder of this network died a decade ago, but it's still his voice and his teachings that are imparted at all of the retreat centers ...warning people that doing [this] practice is like undergoing surgery of the mind, and to leave halfway through is like walking out of an operation before you've been stitched up by the surgeon.
There was one man who said that every time he closed his eyes he could see streams of bubbles everywhere. And he didn't want to leave because he kind of wanted to fix that. and he thought, I might be stuck seeing streams of bubbles forevermore if I leave before the end of this.
At a lot of these centers you also hand in your keys and phone at the beginning, and that's quite an overt cue that you're here for the full period. You can of course go and ask someone and insist that you want them back, but several sources told me that when they expressed a desire to leave, they were pressured not to.
Muraskin: What did your sources –the meditators that experienced harm or their families – think needs to change to make these retreats safer?
Mariage: So first and foremost, warn people before they go in that mental health problems or kind of psychological distress is possible. It's a bit like putting warnings on bottles of medication that, you know, a tiny percentage of people with this prescription might have an adverse effect.
Secondly, they would like to see mental health practitioners on site. So rather than telling everybody to keep meditating, they need to be able to figure out better when somebody needs a bit more support and what that support should be.
Thirdly, they need proper emergency protocols. So for the two women who lost their lives after attending retreats, the horse had already bolted by the time their parents were contacted. I think it needs to be a lot more proactive in terms of reaching out to emergency contacts.
Muraskin: I can imagine you've received some pushback on the podcast from people who've really benefited from Vipassana retreats. What's your response to people who say you've painted the Goenka network too negatively?
Mariage: We've had a couple of emails from people who say this is really one-sided, you're not looking at the positive experiences at all, this has changed my life for the better.
But the podcast isn't about the people for whom this works.... The purpose is to scrutinize harm that is being done to people and to question why isn't the organization itself doing more to prevent that harm.
Andrea Muraskin is a contributor to NPR's Shots blog and writes the weekly NPR Health newsletter. She lives in Boston.
- silent retreats
- goenka retreats
- mindfulness
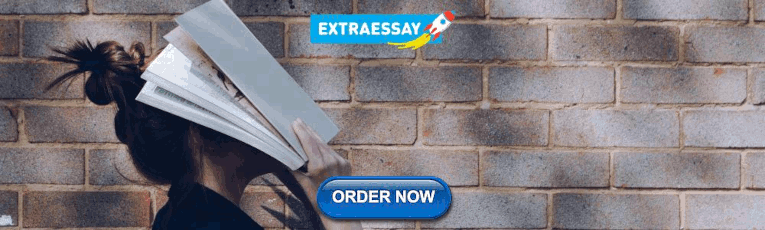
IMAGES
COMMENTS
People who are exposed to sleep loss usually experience a decline in cognitive performance and changes in mood (for meta-analyses, see Pilcher and Huffcutt 1996; Philibert 2005 ). Sleep deprivation is a study design to assess the effects of sleep loss. In acute total SD protocols, the subjects are kept awake continuously, generally for 24-72 ...
Sleep deprivation and daytime sleepiness amongst adolescents and college students cause mood ... Dehydration influences mood and cognition: a plausible hypothesis? Nutrients 3(5):555-573.
Vigilant attention is impaired by sleep deprivation and restored after rest breaks and (more enduringly) after sleep. The temporal dynamics of vigilant attention deficits across hours and days are ...
Key Points. Sleep deprivation triggers a set of bidirectional changes in brain activity and connectivity, depending on the specific cognitive or affective behaviours engaged. Changes in brain ...
The HBM may be a useful model for researchers seeking to predict the negative health outcomes from poor sleep. Knowlden et al. specified a HBM model to measure and predict the sleep behavior of employed college students [18].This cross-sectional study found the HBM explained 34% of the variance in sleep behavior, with perceived severity, perceived barriers, cues to action, and self-efficacy ...
Sleep deprivation is a study design to assess the effects of sleep loss. In acute total SD protocols, the subjects are kept ... performance during sleep deprivation (on lapse hypothesis, eg, Williams et al 1959, see Dorrian et al 2005; Kjellberg 1977). The lapses are caused by microsleeps characterized
Long-term total sleep deprivation: no sleep for more than 48 hours Nocturnal awakenings: waking up during a period of sleep Limitations As with all correlational studies, the findings from the current study cannot reveal causal relationships between sleep deprivation and personal productivity. Another key limitation has been identified.
The impact of sleep deprivation after learning on memory was associated with Hedges' g = 0.277, 95% CI [0.177, 0.377]. ... on studies using total sleep deprivation because depriving a participant of all sleep is a stronger test of the hypothesis that sleep benefits memory than depriving them of a single stage of sleep or restricting their ...
The trace reactivation or replay hypothesis 56,57 suggests that sleep helps memory consolidation through reactivation of traces of ... sleep deprivation leads to lower metabolism in the ...
Sleep deprivation is the restriction of sleep below the level of basal sleep need; this can be both acute (a single period of extended wakefulness), or chronic (the accumulation of sleep debt over multiple nights of sleep restriction). The basal sleep need of an organism is its habitual sleep duration in the absence of pre-existing sleep debt, with sleep debt defined as the duration of sleep ...
the sequential theory of Giuditta (2014) proposes that an interaction between SWS and REM is key. However, all current theories make the ... sleep deprivation aftertwo recovery nightsand even6monthslater. Recent studies havecast doubtonthe privileged statusof thefirst night of sleep, however. Schönauer et al. (2015) found only a short- ...
As in restorative theory, this concept deals with information processing and memory formation. Research suggests that sleep loss leads to less structural plasticity, which can have a negative effect on alertness, cognition, and mood. Sleep deprivation also compromises memory formation, which is related to learning and plasticity.
Sleep deprivation has been shown to negatively affect picture classification speed and accuracy, as well as recognition memory. ... Another theory suggests that the stress brought on by sleep deprivation affects memory consolidation by changing the concentration of corticosteroids in the body.
Previous studies suggest that interference control may be unaffected by sleep deprivation based on the unchanged interference effects (reaction time [RT] differences between incongruent and congruent conditions), while ignoring the overall slower RTs after sleep deprivation. In the present study, we interpreted these results from a new angle using a variant of diffusion model, diffusion model ...
Another important research avenue is the study of chrono-medical timing of sleep deprivation and light exposure for their positive effects on mood in depression. ... the neuroplasticity hypothesis ...
In the long-term, this lack of sleep can come with steep consequences, including an increased risk of car crashes, workplace error, heart problems, reduced immune function, obesity, a lower quality of life, and an earlier death. We explore symptoms of sleep deprivation, the process of being diagnosed, and tips for treating and preventing this ...
Multiple systems in your body may be impacted by sleep deprivation. Research conducted at Penn State found that restricting sleep for 10 days could begin to affect the way your body metabolizes fats.
You have a break at specific times, your day ends at 8, 9 p.m. And then in theory, you go to bed. ... that they ended up struggling to sleep. So they would meditate more because they had initially ...