Edinburgh Research Archive

- ERA Home
- Engineering, School of
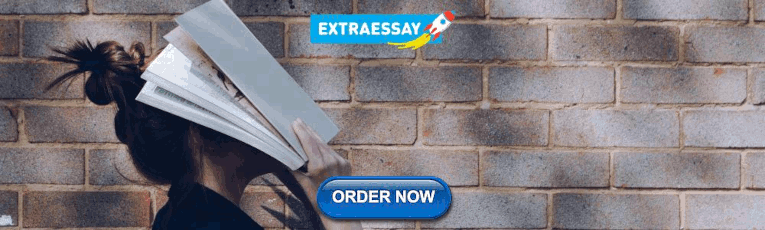
Engineering thesis and dissertation collection

Show simple item record
Underwater optical wireless communications in turbulent conditions: from simulation to experimentation
Files in this item, this item appears in the following collection(s).

- Frontiers in Communications and Networks
- Optical Communications and Networks
- Research Topics
Optical Wireless Communication Technologies
Total Downloads
Total Views and Downloads
About this Research Topic
Optical Wireless Communication (OWC) is a line-of-sight wireless communication system in which data is transmitted in the form of laser beams. OWC is one of the best communication systems because of its high bandwidth, permissionless, highest transfer data rate, and ultra-high security. The development of OWC has increased extensively over the past decade, aided by the availability of powerful system components developed for the field of optical fiber communications. OWCs mainly pass through channels such as free space, the earth's atmosphere, seawater, and interstellar space. However, the channel environment, such as the atmosphere, will seriously affect the wavefront performance of light, causing intersymbol crosstalk and resulting in an increase in the communication bit error rate (BER). Therefore, how to improve the communication performance of OWC in different channels is a focus of researchers. The current research on OWC technology mainly focuses on an advanced optical terminal and photoelectric system fusion design to improve the signal-to-noise ratio of light, using an efficient pointing acquiring and tracking (APT) unit to obtain better beam pointing, and studying the influence of atmospheric turbulence and seawater channels on the performance of light waves for obtaining optimal design parameters. There is also a focus on employing advanced modulation, demodulation and error correction coding technology to get higher transmission rate and lower BER, studying OWC ad hoc network protocols and advanced network topology structure to achieve the purpose of networking applications, and so on. This Research Topic will therefore discuss the related technologies of OWC, so that researchers can better understand the composition of OWC technology, grasp the latest trends in OWC technology development, and achieve the goal of promoting the development of OWC technology. We seek high-quality submissions related to (but not limited to) one or more of the following topics: ● Present and future optical wireless communication terminals, systems and links ● Atmospheric propagation, transmission effects and compensation techniques ● Adaptive optics technology in OWCs ● Advanced optical signal processing technologies ● Optical communication ad hoc network and network topology ● Visible light and underwater optical communication technologies Researchers are encouraged to submit complete unpublished papers, exploring emerging technologies in Optical Wireless Communication.
Keywords : optical wireless communications, optical signal processing, optical communication network, optical propagation, adaptive optics
Important Note : All contributions to this Research Topic must be within the scope of the section and journal to which they are submitted, as defined in their mission statements. Frontiers reserves the right to guide an out-of-scope manuscript to a more suitable section or journal at any stage of peer review.
Topic Editors
Topic coordinators, submission deadlines, participating journals.
Manuscripts can be submitted to this Research Topic via the following journals:
total views
- Demographics
No records found
total views article views downloads topic views
Top countries
Top referring sites, about frontiers research topics.
With their unique mixes of varied contributions from Original Research to Review Articles, Research Topics unify the most influential researchers, the latest key findings and historical advances in a hot research area! Find out more on how to host your own Frontiers Research Topic or contribute to one as an author.
Advertisement
Design and Feasibility Verification of 6G Wireless Communication Systems with State of the Art Technologies
- Open access
- Published: 28 December 2021
- Volume 29 , pages 93–117, ( 2022 )
Cite this article
You have full access to this open access article
- Ravilla Dilli ORCID: orcid.org/0000-0003-0450-8584 1
5786 Accesses
13 Citations
Explore all metrics
Frequencies above 100 GHz are the promising frequency bands for 6G wireless communication systems because of the abundant unexplored and unused spectrum. The increasing global demand for ultra-high spectral efficiencies, data rates, speeds and bandwidths in next-generation wireless networks motivates the exploration of peak capabilities of massive MIMO (Multi–Input–Multi–Output) wireless access technology at THz bands (0.1–10 THz). The smaller wavelengths (order of microns) of these frequencies give an advantage of making high gain antennas with smaller physical dimensions and allows massive spatial multiplexing. This paper presents the design of ultra-massive MIMO (ultra-mMIMO) hybrid beamforming system for multi users and its feasibility to function at THz frequency bands. The functionality of the proposed system is verified at higher order modulation schemes to achieve higher spectral efficiencies using performance metrics that includes error vector magnitude, symbol constellations, and antenna array radiation beams. The performance results suggest to use a particular mMIMO antenna configuration based on number of independent data streams per user and strongly recommended to use higher number of data streams per user in order to achieve higher throughputs that satisfy the needs of 6G wireless systems. Also the performance of the proposed system at 0.14 THz is compared with mmWave systems that operate at 28 GHz and 73 GHz bands to justify the feasibility of the proposed work.
Similar content being viewed by others
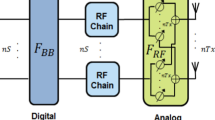
Hybrid Beamforming in 5G NR Networks Using Multi User Massive MIMO at FR2 Frequency Bands
Ravilla Dilli
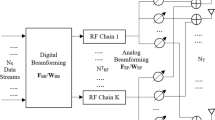
Performance analysis of multi user massive MIMO hybrid beamforming systems at millimeter wave frequency bands
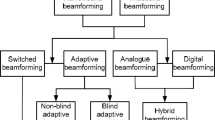
Beamforming techniques for massive MIMO systems in 5G: overview, classification, and trends for future research
Ehab Ali, Mahamod Ismail, … Nor Fadzilah Abdulah
Avoid common mistakes on your manuscript.
1 Introduction
As the 5G wireless technology will be inadequate in the near future due to explosive growth of D2D networks like IoT, IIoT, and IoS. Next generation wireless communication systems, 6G is aimed at supporting communication needs of 2030s. Therefore, 6G wireless technology is essential which uses terahertz (0.1–10 THz) frequencies of EM spectrum and supports 10–100 GHz bandwidths, data rates of Terabits/sec 6G wireless system architecture is an integrated system of different radio access technologies with ultra-massive scales and dynamic network resources to achieve diverse performance improvements. The radio network architecture of 6G cellular systems should make use of cognitive radio, carrier aggregation, THz bands, ultra-mMIMO, and femto cell-based heterogeneous networks to provide maximum capacity [ 1 , 2 , 3 ]. The main aim of this article is to propose and design a hybrid beamforming system for 6G wireless networks that consist of ultra-mMIMO antenna array configurations of size varies from 64 to 256 at BS and 4–16 at MS of multiple users that operates at 0.14THz frequency band. The performance measurements at 0.14 THz are conducted and feasibility of the proposed system is verified for higher order QAM modulation schemes, also compared with mmWave hybrid beamforming systems that operate at 28–73 GHz bands.
1.1 Applications of mmWave and THz Frequency Bands
6G use applications include autonomous driving, industrial automation, machine-to-machine communications that have hard thresholds in terms of throughput, latency, and reliability. There are challenges in 6G system architecture design, network and resource management.
The mmWave and THz applications mentioned in Fig. 1 and Table 1 demand data rates of the order 100 Gbps and wider bandwidths (in terms of GHz) which can be possible with transmission schemes whose minimum spectral efficiencies are 14bits/sec/Hz. Unavailability of such digital modulation schemes and transceiver components to achieve higher data rates in the current scenarios motivates to explore the frequency spectrum above 100 GHz. “Information showers(IS)” provide extreme data rates (> 1Tbps) for ultra-short range wireless networks at THz frequencies. IS makes massive offloading of heavy traffic (up to 95%) in long-range networks and improves UE’s energy efficiency [ 5 ]. In wireless cognition applications, massive computations are conducted on a high capacity remote device like centralized servers. Low power drones (example of resource constrained robots) having limited on-board computing facilities takes advantage of cloud robotics and offload the computations to a cloud server [ 6 ]. In drone based cellular networks (3D), BSs and UEs uses drones to ensure full coverage with less number of BSs in the given space. 3D cellular networks achieve up to 46% reduction in average delay at UEs that includes backhaul delay, transmission, computation delays, also improves spectral efficiency [ 7 ]. GPS based navigation systems are vulnerable to jamming via RF interference, as an alternate THz signals can be used to have high precision range measurements [ 8 ]. Sensing applications mentioned in Table 1 exploit the absorption characteristics of channel materials, frequency-selective channel fading, ultra-mMIMO, and wider bandwidths at THz frequencies. Electronic beam steering techniques and ability of “wireless reality sensing” allows to generate real-time high resolution 3D maps of any given location with less latencies (< 10 nsec). At mmWave and THz frequencies, the effect of ambient light and weather have lesser impact on radar channel than LIDAR. Dual-frequency radars at THz bands give high resolution (similar to high-definition videos) under heavy rain or foggy, cloudy weather conditions whereas LIDAR have very poor performance under such conditions. “Dark vision” is the ability to reconstruct 3D maps of unknown environments at mmWave and THz bands using the combined techniques of sensing, imaging, and position location. Imaging methods at THz bands have less computational complexity in reconstruction of images and less hardware complexity than optical imaging methods even under NLOS channel conditions. THz time-domain high-resolution images can be generated by radar systems for objects irrespective of their shape, size, position and orientation where edges (edge lengths less than 400 µm) are clearly detected [ 9 ]. By exploiting mMIMO beam steering, arrival time/angle of uplink pilot signals one can generate the mmWave image of surrounding environment (both LOS and NLOS objects). Presence of ultra-mMIMO antenna arrays and large available bandwidths at mmWave and THz bands made possible to locate both LOS and NLOS users with centimetre precision [ 10 ].
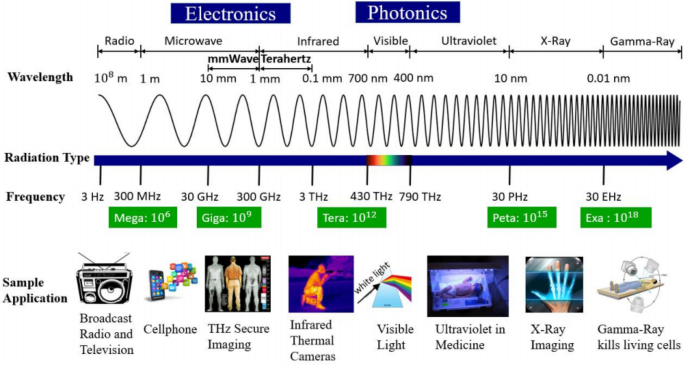
EM spectrum and possible Applications [ 4 ]
Optical wireless communication technologies such as free space optical communication, light fidelity, visible light communication, and optical camera communication can provide effective solutions for deployment of 6G systems and IoT [ 11 ]. Combination of free-space optical and mmWave systems provide significantly high data rates in wireless transmissions in spite of rapid variations in channel and environmental conditions. The selection of active link is determined by index modulation that does not need CSI or any feedback at Tx and it provides higher spectral efficiency [ 12 ]. The combination of optical and digital system architectures at THz bands provide data rates of Terabits/s, nearly zero latency and bandwidths greater than 50 GHz. The front-end transceivers are designed such that they support higher-order modulation schemes to provide ultra-high spectral efficiency [ 13 ].
This paper is organized as follows: Sect. 1 provides insight into mmWave and THz applications. Section 2 explores design challenges, key enabling technologies, role of AI, security aspects in 6G wireless networks. Section 3 describes the wave propagation, channel modelling and measurements at THz bands. Section 4 explores the possibility of using ultra-massive MIMO hybrid beamforming techniques for improved front-end performance at frequencies above 100 GHz. Section 5 discusses the results of ultra-massive MIMO hybrid beamforming system at 140 GHz and compared with the performance at 28 GHz, and 73 GHz mmWave frequencies [ 14 , 136 ]. Section 6 gives overall observations, conclusions and possible future directions of research that can be carried out towards THz frequency bands.
2 6G Wireless Communication Systems
2.1 design challenges and key enabling technologies.
The connectivity goals of 6G wireless systems include: usage of THz frequencies to have abundant bandwidths, pervasive AI, cell-free mMIMO, backscatter communication to save energies, massive network automation etc., Beyond 6G, technologies like internet of nano and bionano things, quantum communications are expected to have impact on wireless communication systems [ 15 ]. Free-space optical network, wireless optical technology, AI, THz, blockchain, quantum communications, 3D networking, driverless vehicles, integrated sensing and communication, cell-free communications, integration of wireless information and energy transfer, backscatter communication, integrated access-backhaul networks, dynamic network slicing, intelligent reflecting surface, holographic beamforming, big data analytics, and proactive caching are the key enabling technologies to support 6G wireless systems development with guaranteed QoS [ 16 ]. In spite of technical challenges, it is possible to implement 6G wireless networks above 100 GHz for the applications shown in Table 1 . Using special theory of relativity, computational complexity of signal processing in adaptive antenna arrays can be minimized and improve the performance of digital phased array antennas. The new path loss, partition loss and channel models (that considers correlation over space and minute changes) are defined for frequencies above 100 GHz.
“Unmanned aerial vehicles (UAV)” communication is one of the important components of 6G wireless technologies where flexible deployment, high degree of design freedom with controlled mobility, strong LOS paths are expected [ 17 ]. UAV-mounted flying BS allow data transmissions to a number of radio devices spread over the area. Optimized trajectories for high throughput and efficient discovery of channel parameters can be designed in UAV communication systems [ 18 ]. UAV communication provides high QoS in deep rural, difficult-to-serve areas, disaster-stricken areas using relay signals. 6G wireless networks are open and intelligent to support dynamic services in IoT which demands for convergence of computing, caching and communicating (3C). 6G wireless technologies support 3C-based spectrum management, wireless distributed computing, delay-aware transmission and network selection [ 19 ]. Deployment of smart health-care systems using 5G wireless technologies have challenges in terms of device density, latency, bandwidth, security, and energy efficiencies [ 20 ]. It is important to focus on study of channel, devices, and space-based system at mmWave and THz frequencies [ 21 , 22 ]. 6G wireless technologies are the key for IoE applications that integrates applications ranging from virtual reality to autonomous systems [ 23 ]. 6G wireless technologies are expected to have high energy efficiency and technical standard of new spectrum to address the challenges including network designs, testbed developments, physical payer transmissions, and security [ 24 ]. High data rates can be achieved at THz bands such as 0.275–3 THz, however there are challenges in designing adaptive transceivers (both RF and digital domain), signal processing algorithms, channel characterization, privacy, and security [ 25 ].
6G wireless network features include high-precision 3D localization, ultra-low latency, high reliability, and high bandwidths leads to native applications like distributed, ubiquitous AI, IoT. In 6G wireless networks, the key enabling technologies such as ultra-mMIMO, THz, a tactile internet should address security aspects in 6G [ 26 ]. The design and development challenges in high-speed T–R modules, ultra-wideband antennas, channel modelling, higher order modulation schemes at THz bands are the new frontiers of 6G wireless systems [ 27 ]. Investigation of nonlinear optical effects and nanoscale phenomena at THz frequencies are essential as THz bands play an important role in 6G wireless networks [ 28 ].
2.2 Role of AI in 6G Wireless Networks
AI and machine learning techniques are most important features in making intelligent 6G networking systems [ 29 ]. AI plays a crucial role in 6G network designs and protocols to support mobile internet as well as ubiquitous AI services for the users [ 30 ]. Next generation driving use cases (vehicular communication: vehicle-to-everything applications) are AI based which is an emerging field in 6G vehicular networks that demands high reliability, ultra-low latency, high data rates, and high security [ 31 , 32 ]. To address these use cases, mmWave mMIMO beamforming techniques are essential with beam alignment and beam sweeping capabilities. Also, optimal beam sweeping schemes have to be introduced at Tx and Rx to reduce the latency in achieving beam alignment [ 33 ]. AI-powered applications demand 6G wireless technologies and motivates to in-depth research possibilities in terms of coverage, spectrum, energy consumption, air interface, and delay [ 34 ]. Applications of 6G wireless networks can get potential benefits with the synergies of quantum computing, machine learning and deep learning techniques [ 35 ].
AI based architecture for 6G includes flexible radio access network-slicing, mobile edge caching & content delivery, and automated radio access technology selection [ 36 ]. Air-interface designs (integration of communication with radio sensing) in 6G wireless technologies are optimized by AI techniques to meet the latency, reliability and synchronization requirements [ 37 ]. AI-enabled 5G and 6G cellular networks deployment provides significant improvements in performance and robustness at lower complexity [ 38 ]. AI-based adaptive scheme for 6G IoT networks provides security where IoT devices are connected to mobile networks using different THz and mmWave frequency bands. Extended Kalman filtering in 6G networks can estimate future harvesting power and avoid energy exhaustion [ 39 ]. Deep learning-based (using a deep neural network structure) mmWave beam selection in 5G NR by exploiting sub-6 GHz channel information minimizes beam sweeping overhead by 79.3% [ 40 ]. Heterogeneous multi-layer mobile edge computing in which MSs generate raw data of computing tasks in radio access networks to cloud centre that involves collaborative data processing at various network layers. It is essential to have ultra-low latency service in 6G networks which is possible by reinforcement learning in pricing scheme design, network congestion control, cognitive radio access, and task offloading for volatile wireless environments [ 41 ]. 6G mobile network architecture is expected to operate at THz frequencies with high QoS support and energy efficiency. The architectural challenges of 6G networks include 3D coverage, efficient protocol stack, pervasive AI [ 42 ]. AI (deep learning methods) techniques optimize the performance of space-air-ground integrated networks by improving traffic balancing [ 43 ]. Hybrid beamforming designs for mMIMO with minimum number of RF chains and phase shifters based analog beamforming designs are challenging with imperfect CSI [ 44 ]. “Multitask deep learning” techniques are used in hybrid beamforming for MU-mMIMO OFDM systems [ 45 ]. Deep learning based beamforming designs maximizes spectral efficiency with imperfect CSI and less hardware.
2.3 Security Aspects in 5G and 6G Wireless Systems
It is challenging to implement security in 5G and 6G wireless networks as they are deployed in decentralized, heterogeneous, and massive ubiquitous devices. Blockchain technology can provide security and privacy for 5G and 6G networks like edge computing, cloud computing and device-to-device communications [ 46 , 47 ]. 6G applications scenarios demand for high privacy, secrecy, and security [ 48 ]. Physical layer level security techniques are essential at THz frequencies to address threats like eavesdropping which is possible by placing an object and intercept the LOS signal to get scatter radiation in the direction of eavesdropper. However, characterization of backscatter in channel addresses the problem of eavesdrop and also detects eavesdropper [ 49 ]. Security can be provided in mmWave mMIMO wireless systems using channel sparsity where the data is precoded onto dominant angle components while artificial noise in broadcasted over non-dominant angles which interfere with eavesdropper’s signals. The entropy of channel sparsity (is unknown to eavesdropper) defines secrecy rate and optimal levels of sparsity provides maximum secrete rate [ 50 ].
3 Wave Propagation at THz Frequencies
3.1 wave propagation scenarios (reflections, diffraction, scattering).
The radio signal propagation undergoes through three main propagation mechanisms called reflection, diffraction and scattering in multipath channel as shown in the Fig. 2 [ 51 ]. At THz carrier frequencies, signal transmissions are highly directive (less angular divergence) and effects of diffraction is minimum. Adaptation of mMIMO, hybrid beamforming or beam steering techniques overcomes the shadowing effects at mmWave frequencies [ 52 ].
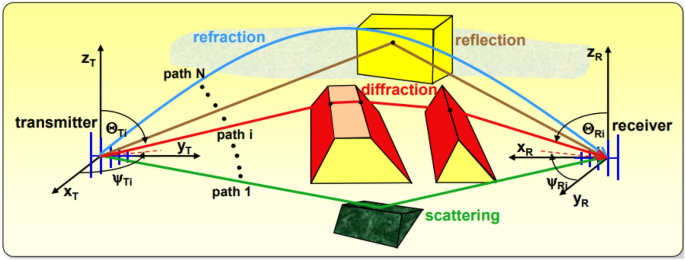
Wave propagation in a multipath fading channel
Scattering is an important propagation mechanism to consider in channel modelling at THz frequencies for 6G wireless systems. The surface scattering at THz frequencies exhibit both diffuse and strong specular reflections. “Directive scattering” and “radar cross section” scattering models support development of ray tracing tool design, imaging, and localization for 6G wireless systems [ 53 ]. Characterization of surface scatterers (for both diffused and specular components) at 60 GHz leads to small-scale fading due to rough surface scatterers. The diffused scattering depends on distance from the surface, angle of incidence, and material roughness [ 54 ]. The multipath delay spread and path losses of a radio channel are characterized at frequency range 126–156 GHz [ 55 ].
Wavelengths of THz frequencies approach the dimensions of dust, snow particles or rain and effects of Mie scattering becomes more significant contribution to link budget. The rain effects do not contribute to additional attenuation losses at THz frequencies (0.1–0.5 THz) [ 56 ]. Atmospheric gases like hydrogen, oxygen, and carbon-di-oxide etc., causes significant attenuation at THz bands (183 GHz, 325 GHz, 450 GHz, 550 GHz, 760 GHz) and these frequency bands can be used for secure ultra-short range communications [ 57 , 58 ].
3.2 Channel Modelling and Measurements
Channel properties define the performance limits in 6G wireless communication system. So, it is very important to explore different 6G channel models, measurement techniques and develop statistical channel impulse response models both in space and time at THz frequencies [ 59 , 60 , 61 ]. 3GPP defines human blockage, spatial consistency, and outdoor-to-indoor penetration losses as main components of channel modelling for mmWave mMIMO hybrid beamforming system designs. A geometry-based, spatially consistent channels are realized by generating time-variant and spatially correlated channel coefficients. Human blockage shadowing losses are modelled at 73 GHz using four-state Markov model [ 62 , 63 , 64 , 65 , 66 ]. Radio propagation models or channel models (both large-scale, small-scale fading, also MIMO channels) for mmWave 5G wireless systems are defined with hybrid beamforming and coordinated multi-point transmissions at 24 GHz to 86 GHz frequencies. The performance metrics (coverage, spectral efficiency, hardware requirements) are highly dependent on channel models [ 67 ]. mmWave technology provides adaptive tuning in military communication networks as per atmospheric variations and high node mobility without compromise for high data rates [ 68 ]. Various channel models and propagation parameters of 5G wireless communication systems such as building penetration losses, large-scale path loss, LOS probabilities helps to understand the propagation of mmWave frequencies over 0.5–100 GHz [ 69 ].
In the presence of CSIT, antenna array gains and spatial multiplexing gains are fully utilized in mMIMO systems. But, conventional CSIT techniques do not suit FDD mMIMO systems due to their feedback and training overhead. Three pilot training strategies are proposed for acquiring CSI in MU mMIMO mmWave systems using “generalized block OMP algorithm” that performs better than conventional OMP algorithm [ 70 ]. Joint optimization technique of communication and computation powers is formulated for MU mMIMO systems with partially-connected RF structures that saves powers up to 76.59% [ 71 ]. “Generalized-block compressed sampling matching pursuit algorithm” offers better performance in acquiring CSI and minimizes errors compared to “generalized block OMP algorithm” [ 72 ]. Using a distributed compressive sensing CSIT, feedback and training overhead can be minimized in MU mMIMO hybrid beamforming systems [ 73 ].
Requirement of high data rates and bandwidths for smart rail mobility motivates to explore high gain mMIMO, advanced handover designs, and dynamic beamforming techniques at mmWave and THz bands [ 74 ]. The communication between passengers and rails as well as intra-wagon scenarios are characterized using ultra-wideband channel sounding and ray tracing at 60–300 GHz frequency bands with 8 GHz of bandwidth [ 75 ]. The measured propagation losses of a communication link at 350 GHz frequency estimates the data rates of 1Gbps at 8.5 m and 100Gbps for 1 m distances [ 76 ]. Channel models and propagation measurements are presented at D-band (110–170 GHz) frequencies. Channel sounder system is designed for 140 GHz and signal attenuation measurements are investigated for indoor models [ 77 , 78 ]. The large-scale path loss and multipath time dispersion measurements for ultra-dense indoor wireless networks at mmWave bands show that multipath RMS delay spread can be minimized using more directive antennas [ 79 ]. Certain mmWave and THz frequency bands exhibit less path loss (< 10 dB/Km) in addition to free-space path loss values. Therefore, these frequencies can be used for 6G cellular and mobile applications (long-range communications) [ 80 ].
THz frequencies exhibit a very high signal attenuation or path loss, however, we can overcome this using ultra-mMIMO antenna arrays which compensate increased path loss and provides highly reliable links. Neighbour discovery is challenging in mMIMO systems at THz bands and this process can be made time-efficient by using the complete antenna radiation pattern instead of only main-lobe [ 77 , 81 ].
3.3 Channel Measurements at 73 GHz
Indoor wave propagation mechanisms (scattering, reflection, path-loss) and channel properties are studied at mmWave and Terahertz (28 GHz, 73 GHz and 140 GHz) frequencies. At these frequencies, reflection coefficient is linearly increasing with increase in angle of incidence and the scattered power is 20 dB less than the reflected power. Also, for a given angle of incidence, path loss is inversely proportional and partition loss (material dependent) is proportional to frequencies [ 82 ]. mmWave multipath signal propagation characteristics for indoor communication were measured in terms of power received, path loss and delay spread at 28 GHz, 39 GHz, 60 GHz, and 73 GHz frequencies. The received power and delay spread are inverse in relation to T–R separation, whereas path loss is proportional to T–R distance. The amount of path loss due to multi-floors is very high at 28–39 GHz compared to 60–73 GHz [ 83 ]. Coordinated Multipoint and BS diversity measurements of a large-scale mmWave 5G wireless system at 73 GHz shows the improvements in interference suppression where 43% of users overcome interference [ 84 ]. Penetration losses, outdoor foliage induced scattering, and ground reflection measurements are conducted at 73 GHz, 81 GHz with both cross-polarized and co-polarized antenna configurations to provide consistency and reliability in measurements at any given frequency [ 85 , 86 , 87 , 88 ].
Beam steering capabilities of directional antenna arrays at mmWave bands can overcome the path loss of a multipath channel in a dense urban cellular environment [ 89 ]. Large-scale path loss models based on ultra-wideband mmWave propagation measurements at 28–73 GHz offer simplicity in path loss calculations and predictions with high accuracy. 3GPP floating-intercept path loss model needs a simple modification for converting in to the close-in free space reference distance models [ 90 ]. Indoor and outdoor channel measurements at 100 GHz, 200 GHz, 300 GHz, and 400 GHz are carried out for a link data rate of 1Gbps. The multi-path channel measurements proved the feasibility of using THz radio links for data transmissions in a highly fading channel environments [ 91 ]. The radiation at THz (0.1–10 THz) bands penetrates through materials (indoor office) with minimum penetration losses and loss increases for higher end of THz band [ 92 ].
4 Ultra-mMIMO and Hybrid Beamforming Techniques for 6G
mMIMO and ultra-mMIMO are the key enabling technologies in 5G and 6G networks respectively with efficient beam steering capabilities. The important open research challenges in mMIMO system design are channel estimation, pilot contamination, user scheduling, precoding, signal detection, and energy efficiency [ 93 ]. The mMIMO and ultra-mMIMO beamforming systems offers huge bandwidths with very high data rates in 5G and 6G cellular mobile systems. They provide maximum antenna array gains by overcoming the high path loss, atmospheric absorption, and rapid channel variations. These designs demand precise alignment of BS and UE beams that increases latency in link establishment, implications of control layer procedures like beam tracking, and handover [ 94 ].
6G wireless technologies use ultra-mMIMO antenna arrays and THz frequencies to support AI-infused applications, autonomous driving, IIoT, wireless brain–computer interaction, wireless backhaul, augmented/virtual reality, and holographic applications [ 95 ]. Design and development of RF antenna systems for beyond 5G and 6G are very important as the operating frequencies are very high. It is important to focus on breakthrough performance of antenna system with new materials and metamaterials, evolution of propagation channels, and updates in antenna measurement technology [ 96 ]. ultra-mMIMO communications can overcome the higher propagation losses at THz bands and increase the communication range. Graphene-based plasmonics allow us to design large antenna array structures within small area (e.g., 144 antenna elements per 1cm 2 at 60 GHz, 1024 antenna elements per 1 mm 2 at 1 THz) and enables ultra-mMIMO communication in 6G wireless systems [ 97 ]. “quantum-inspired social emotional optimization” algorithm achieves optimal power control and QoS in mMIMO UL networks for maximizing spectral efficiency [ 98 ]. Accuracy of beam selection, beam pair update delay effects the QoS performance. Best measurement techniques for mobility and beamforming management needs to be followed to have more accuracy in 3GPP NR mmWave systems [ 99 ]. In a MU mMIMO systems, low complex solutions are proposed to reduce Tx power based on individual SINR constraints for users less than and greater than the number of RF chains [ 100 ]. Low complex inter-user interference reduction algorithms are used in MU mMIMO UL systems to achieve performance close to fully digital beamforming system [ 101 ].
To minimize the hardware cost of MU mMIMO systems, “hybrid beamforming with selection” considers instantaneous channel variations using switch bank architecture [ 102 ]. Joint user scheduling is proposed for MU mMIMO OFDM systems where users with identical radiation beams are multiplexed using OFDM to improve the system performance [ 103 ]. Accuracy of channel estimation in MU mMIMO systems is improved using its continuous representation instead of grid-based as a result there is an improvement in spectral efficiency [ 104 ]. A novel hybrid beamforming design is proposed to improve the total sum rate based on “weighed sum mean square error minimization” and “compressed sensing” under perfect CSIT [ 105 ]. “Manifold optimization”, “eigenvalue decomposition”, and OMP hybrid beamforming algorithm is proposed for mmWave systems to minimize the computational complexity, mean square error and increase transmission reliability as well as spectral efficiency [ 106 , 107 ]. Relay assisted MU mMIMO hybrid beamforming mmWave system can overcome the path loss and improves system performance [ 108 ]. Splitting of hybrid precoding and combining between Tx and Rx along with OMP algorithm increases the total sum data rates in a MU mMIMO systems [ 109 ]. In MU mMIMO hybrid beamforming systems, under imperfect CSI, radiated power of UEs is inversely proportional to square-root of the number of BS antennas whereas with perfect CSI, radiated power is just inversely proportional to number of BS antennas [ 110 ]. If the number of BS antennas are minimum, MRC performs better than ZF and as number of BS antennas are increasing, uplink data sum rates for MRC and ZF becomes equal. Total data sum-rate is maximized by minimizing weighted sum MSE (based on block diagonalization in digital beamforming) in DL MU-mMIMO hybrid beamforming systems with perfect CSI. Lower capacity bounds for MRC, ZF and MMSE detection are derived. Generally, ZF and MMSE performs better than MRC Rx due to their ability to cancel intra-cell interference. In multi-cell environment, at lower power levels, cross-talk introduced by inferior MRC falls below the noise level and this Rx becomes a viable option. For example, in a small-scale Rayleigh fading channel, 50 MSs can be served with 100 an antenna array of 100 at BS using same time–frequency resources. Therefore, each MS has 1bits/channel/MS and it leads to a total system throughput of 50bits/channel/MS.
mmWave mMIMO DL hybrid beamforming with geographically distributed cluster antennas over the coverage area (remote antenna arrays) decreases the average distance between UEs and arrays, also provides more spatial diversity. This design has performance same as fully digital beamforming in terms of spectral efficiency per user when the number of RF chains are not less than twice the number of UEs [ 111 ]. mmWave mMIMO lens antenna array reduces the number of RF chains considerably in the presence of CSI. “Approximate message passing learned prior-aided Gaussian mixture” beamspace channel estimation algorithm can give more accuracy [ 112 ].
4.1 MU-mMIMO Hybrid Beamforming
In a multi-user communication, multiple users share the same channel, therefore communication needs to be coordinated in T–F domains. In MIMO systems, the Tx power is divided and provide both diversity gain as well as antenna array gain. MIMO beamforming improves spectral efficiency and coverage is high in a particular direction. Hybrid beamforming can be categorized depends on average or instantaneous CSI where instantaneous CSI provides better SNIR and average CSI gives lower overhead in CSI acquisition. Therefore, algorithm selection to get best trade-off essentially depends on channel characteristics [ 113 ]. CSI availability at Rx (CSIR) is collected using pilot signals and CSI at Tx (CSIT) is collected using feedback channels from Rx to Tx. If we have CSIT, the Tx signal can be precoded to provide diversity gain at the Rx, on the other hand if we have CSIR then it is possible to provide diversity using “space–time coding”. MU-MIMO provides all-to-all communication (between K-access points and K-UEs where each UE has multiple independent data streams) and facilitates higher spectral efficiency (over mMIMO) using an interference cancellation beamforming algorithm. Shannon’s limit for channel capacity is defined with K > 3 under Rayleigh fading channel conditions and defined the upper limit for MIMO antenna array size to have an optimum throughput and overhead [ 114 ]. Coordinated multipoint techniques in a multi-cell MU-mMIMO hybrid beamforming system can mitigate intra-cell and inter-cell interference, also achieves high spectral efficiency by maximizing signal-to-leakage-plus-noise ratio [ 115 ].
Data processing steps in a MU mMIMO systems are shown in Fig. 3 where the source encoded user’s data symbols are encoded (channel encoding) using convolutional codes at the Tx. The channel encoded bit stream is mapped to QAM symbols and the resultant QAM data of every user is split into multiple independent data streams. Precoding weights (computed using HBPS algorithm) are assigned for each subcarrier of independent data stream at Tx. The same precoding weights are used to derive the corresponding combining weights at Rx. HBPS algorithm provides all digital beamforming weights and recognize peaks to form corresponding analog beamforming weights. The digital precoded signal is modulated using OFDM with pilot mapping followed by analog beamforming process at Tx antennas. The transmitted signal travels through a scatter rich MIMO channel and this signal is demodulated, and decoded at Rx. Channel sounding and estimations processes are performed using JSDM algorithm that permits large scale MIMO antenna arrays at BS with less CSI feedback information from UEs of a mMIMO DL channel.
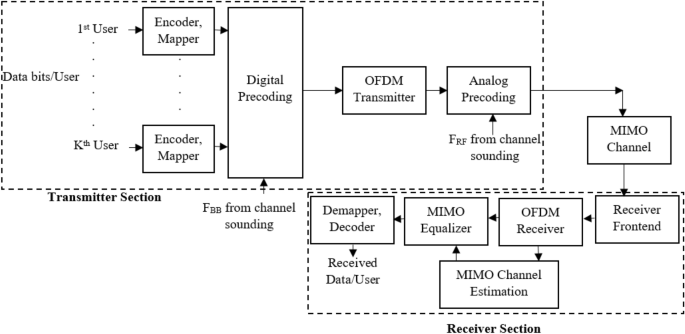
Data processing steps in a MU-mMIMO hybrid beamforming system
4.2 Wideband mMIMO-OFDM Systems
OFDM technique eliminates ISI by distributing high-rate user’s data across many number of narrowband sub-channels (those are closely spaced) to provide reliable broadband communication. When the bandwidth is sufficiently large, the channel between any Tx antenna and Rx antenna will appear dispersive. Because of this, we can combine MIMO with OFDM for frequency selective channels as shown in Fig. 4 . We can perform this by connecting OFDM Tx to each transmitting antenna and OFDM Rx to each receiving antenna, and process the signals across different antennas.
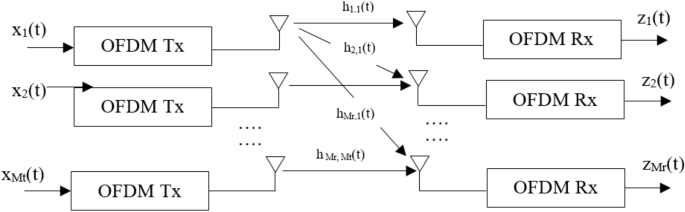
mMIMO-OFDM system
Using MIMO-OFDM, we can convert wideband mMIMO channel into many parallel narrow band MIMO channels. All practical mMIMO systems operate in a wideband region, therefore, mMIMO can be combined with OFDM for frequency selective channels. The combination of MU mMIMO-OFDM gives extremely high spectral efficiencies even in the absence of CSIT. In the presences of CSIT, channel capacity of MU mMIMO-OFDM systems approach the theoretical limits and these systems are essential for beyond 5G wireless communications.
4.3 Channel Modelling in MU-ultra-mMIMO Systems
The mMIMO channel can be characterized as
The channel matrix, \({\mathbf{H}} = \left[ {\begin{array}{*{20}l} {h_{11} } \hfill & {h_{12} } \hfill & \ldots \hfill & {h_{{1{\text{Mt}}}} } \hfill \\ {h_{21} } \hfill & {h_{22} } \hfill & \ldots \hfill & {h_{{2{\text{Mt}}}} } \hfill \\ \ldots \hfill & \ldots \hfill & \ldots \hfill & {h_{{3{\text{Mt}}}} } \hfill \\ {h_{{{\text{Mr}}1}} } \hfill & {h_{{{\text{Mr}}2}} } \hfill & \ldots \hfill & {h_{{{\text{MrMt}}}} } \hfill \\ \end{array} } \right]\) .where h ij is a gaussian complex random variable which models fading gain between jth Rx antenna and ith Tx antenna.
For any given subcarrier ‘k’, the vector observation is given as,
where y → Rx column vector of size M r × 1, H → channel matrix of size M r × M t , X → Tx column vector of size M t × 1, n → AWGN noise column vector of size M r × 1, z k → observation vector of size M r × 1.
High accurate and low complex channel estimation scheme called “Step-length optimization-based joint iterative scheme” is developed for MU mMIMO system that uses less number of training sequences even for large number of users [ 116 ]. Hybrid channel estimation is proposed where the channel matrix is decomposed into an angle matrix and gain matrix. This technique improves channel estimation accuracy with minimum training overhead in a MU mMIMO hybrid beamforming systems [ 117 ]. Lens antennas array based MIMO needs less number of RF chains as it exploits the property of angle-dependant energy focus. “Path delay compensation” techniques that exploits multi-path sparsity converts MU MIMO channels that are frequency selective into parallel narrow-band flat fading channels [ 118 ]. Optimizing hybrid beamforming design of relay system with full-connected and mixed structures maximizes the sum rate in a mMIMO systems [ 119 ].
The changes in angles of arrival/departure (AoAs/AoDs) are less compared to their path gains in a MU mMIMO systems. MSE minimization of received signal using the solution of digital beamforming gives maximum sum rates in a mmWave hybrid beamforming system. The sum rate increases with number of paths (accuracy of AoA/AoD) and number of RF chains [ 120 ]. The estimation of AoA/AoD helps in pilot beamforming and maximizes it power to achieve accurate path gains [ 121 ].
4.4 Channel Estimation in MU-ultra-mMIMO Systems
Estimation of more channel coefficients in a MU-mMIMO systems and high propagation loss in mmWave transmission give a challenge in channel estimation for DL. The BS antenna array uses imperfect CSI that is derived using transmitted pilots signals to extract individual data streams. The radiated power of MSs is inversely proportional to square-root of number of BS antennas with no reduction in performance. If perfect CSI is available, transmitted power at MSs can be made inversely proportional to number of BS antennas. For complete utilization of spatial multiplexing gains (or antenna array gains) of mMIMO, CSI must be obtained at the Tx side.
Joint design of digital and analog stages overcomes loss of information at each stage and this gives optimal solution for MU-mMIMO hybrid beamforming system whose sum rate approaches channel capacity [ 122 ]. The users are grouped into clusters based on feedback and CQI of beams in a mmWave MU mMIMO DL NOMA system which offers maximum sum rates [ 123 ]. Angular representation of channel using non-zero sparse matrix to form clusters in a MU-mMIMO systems better than the system without clusters [ 124 ]. “compressive sensing” techniques reduce the channel measurement complexity and configure hybrid precoders & combiners in a mmWave MU MIMO system with frequency selective channels [ 125 ]. Usage of “penalty dual decomposition” method in hybrid precoding approaches the fully digital precoding performance even in the presence of less number of RF chains at Tx and Rx [ 126 ].
Diagonalization of channel matrix provides unconstrained optimal precoding weights by taking the first N T RF dominating modes under known CSI conditions. Most of the time, CSI is at Rx (CSIR) is acquired using pilot signals which carry channel estimation information. Sometimes, using feedback channels from Rx to Tx we can also collect CSI at Tx (CSIT). If we have CSIR, we can provide diversity gain using “space–time coding”, on the other hand, if CSIT is available, we can precode the transmitting signal to provide diversity gain at the Rx. Under the availability of CSI at BS, MU precoding is performed to transmit signals to all users using same T–F resources and UEs can recover signals with minimum complexity [ 127 ].
Design of two stage beamformers for FDD MU mMIMO DL hybrid beamforming systems optimizes hybrid precoding to increase sum rates and conditional average signal-to-leakage-plus-noise ratio [ 128 ]. Optimal hybrid precoding and combining algorithms based on the principle of basis pursuit demands only low cost RF hardware in mmWave mMIMO systems [ 129 ]. Performance of iterative hybrid precoding approaches the capacity of fully digital precoding scheme [ 130 ]. “Hybrid regularized channel diagonalization” based hybrid beamforming design uses low-complex non-iterative process in a MU-mMIMO DL mmWave channel [ 131 ]. A low-complex hybrid precoding scheme called phased-ZF can approach the performance of full-complexity baseband ZF precoding in a mMIMO systems [ 132 ].
Closed-form expressions are defined to configure mobility-aware hybrid precoder for high-speed mobile users in mmWave mMIMO systems with reduced computational complexity. The number of RF chains can be activated adaptively based on channel conditions to maximize energy efficiency and beamforming gains at high SNRs [ 133 ]. Transform domain precoder is designed for a mmWave mMIMO hybrid beamforming system that reduces 60–89% of overhead and enhances 2.38–21% of spectral efficiency [ 134 ]. Fixed phase shifter implementation in mmWave hybrid precoding is hardware-efficient as it needs only less number of phase shifters with fixed and quantized phase values. A switch network (group-connected mapping) between phase shifters and antennas provides dynamic connections according to channel conditions. This setup maximizes the spectral efficiency with minimum hardware complexity [ 135 ].
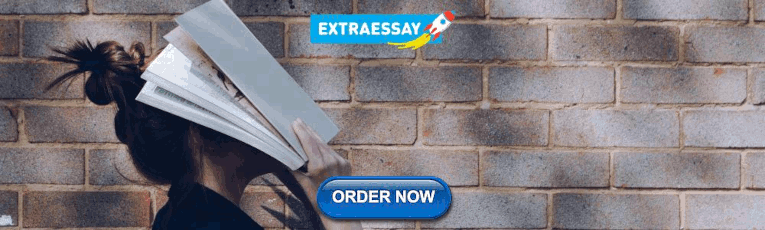
4.5 CSIR Detection Algorithms in MU-ultra-mMIMO Systems
Lower capacity bounds for MRC, ZF and MMSE detection are derived. ZF and MMSE performs better than MRC Rx due to their ability to cancel intra-cell interference. In multi-cell environment, at lower power levels, cross-talk introduced by inferior MRC falls below the noise level and this Rx becomes a viable option. For example, in a small-scale Rayleigh fading channel, 50 MSs can be served with an antenna array of 100 at BS using same T–F resources. Therefore, each MS has 1bits/channel/MS and it leads to a total system throughput of 50bits/channel/MS. In this case, Tx does not know the channel instead only Rx is assumed to know the channel. Therefore, Tx sense the vector x QAM symbols, and Rx observes y . Here, the objective is to recover x from y as \({\mathbf{y}} = {\mathbf{Hx}} + {\mathbf{n}}\) .
4.5.1 ML Detector
It is an optimal detector in terms of error probability in the presence of IID gaussian noise at the Rx. We can find the best possible x by maximizing likelihood from all possible likelihoods as
Since the noise is gaussian, the estimation of x is equal to minimum of Euclidean distance between the observation y and HX for all possible x .
However, the complexity of ML detector increases exponentially with number of Tx antennas, M t .
4.5.2 ZF Detector
ZF equalizer uses inverse of channel frequency response to restore the signal at the Rx and it gives zero ISI in a noise free channel conditions. We can achieve flat fading by combining channel frequency response with ZF equalizer frequency response. This equalizer is essential when ISI is dominating the noise. For mMIMO systems, it is used when M r ≥ M t , and there exists at least ‘M t ’ linearly independent columns in channel matrix, H . It has lower complexity than ML detector. ZF detector equalizer is given as,
where \(\left( {{\mathbf{H}}^{{\text{H}}} {\mathbf{H}}} \right)^{ - 1} {\mathbf{H}}^{{\text{H}}}\) is a pseudo inverse.
If H H H is a singular matrix, then the noise enhancement w is very high. We can perform element by element detection as z 1 = x 1 + w 1 , z 2 = x 2 + w 2 , etc., ZF equalizer can provide higher data rate gains at the cost of diversity gain. However, the challenge in ZR detector is that it needs infinitely long impulse response and also, it can amplify the noise if minimum singular value of channel matrix is too small.
4.5.3 MMSE Equalizer
In MMSE equalizer, we do not exactly recover x instead its approximation, and w noise enhancement value less. MMSE relies on Gaussian model of x . In order to avoid the noise enhancements ( w ), there is a trade-off achieved by MMSE equalizer. MMSE estimate of the transformed symbols x (where x is assumed to be Gaussian) is
where I Mt / ρ is a regularization term that depends on SNR, ρ .
If SNR is too high and approaches ‘∞’ or noise approaches zero, then MMSE approaches ZF detector. In MU-mMIMO systems, favorable propagation conditions in NLOS relies on orthogonal columns of UL channel matrix \({\mathbf{H}}^{{\text{H}}} {\mathbf{H}} \approx {\text{M}}{\mathbf{I}}_{{\text{K}}}\) , rayleigh fading entries from CN (0,1), and scaling of off-diagonal elements. The condition for LOS orthogonality is given as,
4.6 CSIT Detection Algorithms in MU-ultra-mMIMO Systems
FDD mode of channel estimation utilizes different spectrum for UL and DL. In FDD mode, the channel estimation time is proportional to number of antennas and makes the time overhead prohibitively expensive for mMIMO systems. Therefore, we have assumed the channel estimation by reciprocity, so the channel is operated in TDD mode where the same spectrum is used for both UL and DL. UL represents a “standard MIMO” communication system, so we can apply standard detection MIMO detection techniques like ZF, MMSE, maximum likelihood etc., For UL, no CSIT is needed at the users (Txs) for BS to recover the data and processing can occur in one coherence interval. UL can be trained using (B c *T c ) samples with a fixed channel where B c is coherence bandwidth, T c is coherence time. If we use a fraction a for pilots, then the rate (1 − a) x maximum rate. We want to have full spatial reuse, so some users will have same pilot. If any two users have same pilot,
w is noise that depends on length of pilot or power.
Leads to wrong precoder, loss in rate: SINR in UL and DL are interference-limited which is called “pilot contamination”.
4.6.1 Parallel Decomposition
As shown in Fig. 5 , any given complex Mr x Mt matrix can be expressed as \({\mathbf{H}} = {\mathbf{U}}\Sigma {\mathbf{V}}^{{\text{H}}}\) .where U → unitary matrix of size M r x M t , Σ → Diagonal matrix of size M r x M t and rank R H ≤ min(M r , M t ), V → unitary matrix of size M t x M t .

Parallel decomposition using SVD
R H = min (M r , M t ) in a rich scattering environment like Rayleigh fading environment, the value of R H is typically small in a LOS channel.
The estimated symbols,
where, x l → data symbols, x → transformed symbols, y → received symbols.
It creates R H parallel channels out of which no data is transmitted on M t –R H channels. It can be combined with rate and power adaption. This is a simple detector and not affected by noise.
4.7 Capacity of MU-ultra-mMIMO Beamforming Channel
In a MIMO system, transmitting one symbol over all M t antennas leads to high diversity but with low rate. But, in a mMIMO system with beamforming as shown in Fig. 6 gives both diversity gain as well as higher data rates. The received symbols in such systems is given as
where x, y, n are scalars and select U and V along largest singular value.
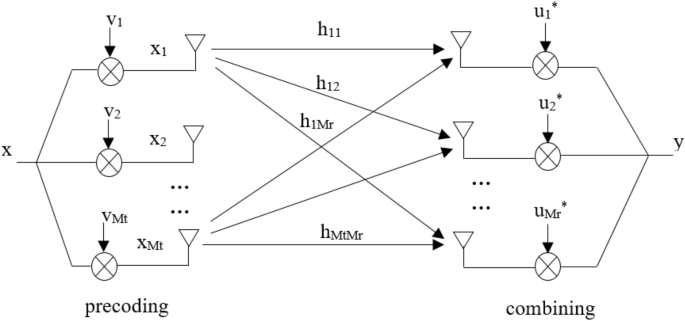
mMIMO channel with beamforming
The narrowband MIMO channel can be represented as,
where \({\text{E}}\left\{ {{\mathbf{xx}}^{{\text{H}}} } \right\} = {\mathbf{R}}_{{\text{x}}} ,\) R x → input covariance matrix and R x T = ρ.
\({\text{E}}\left\{ {{\mathbf{nn}}^{{\text{H}}} } \right\} = {\mathbf{I}}_{{{\text{Mr}}}}\) where n is zero mean gaussian noise.
The channel matrix, H can be represented as \({\mathbf{H}} = {\mathbf{U}}\Sigma {\mathbf{V}}^{{\text{H}}} \,{\text{with}}\,\Sigma = {\text{diag}}\left( {\sigma_{1}^{2} ,\,\sigma_{2}^{2} ,\, \ldots \sigma_{{{\text{RH}}}}^{2} ,\,0,\, \ldots 0} \right)\) .
Channel capacity for fixed channel,
The above equation maximizes overall possible covariance matrices that satisfy the total energy constraint. If the channel is unknown at the Tx (no CSIT), then the total energy is spread among all M t Tx antennas, therefore \({\mathbf{R}}_{{\text{x}}} = \left[ {{\rho \mathord{\left/ {\vphantom {\rho {{\text{Mt}}}}} \right. \kern-\nulldelimiterspace} {{\text{Mt}}}}} \right]{\mathbf{I}}_{{{\text{Mt}}}}\) .
Channel capacity,
On the other hand, if CSIT is available, we can do more sophisticated detection using SVD and exploit power allocation.
In MU-mMIMO systems users are always orthogonal and favourable propagation conditions in LOS relies on geometry of the array and far field conditions. If ‘M’ antenna elements spaced at d = λ/2 and incoming signal from angle ‘θ’, then the path difference \(\delta = {\text{d}}\sin \left( \theta \right)\) . Therefore, the received passband signals are given as follows:
and the corresponding received baseband signals:
Channel (with g k being a complex number, same for all antenna elements)
This allows beamforming in specific directions (d = λ/2).
5 Results and Discussions
3GPP recommends 28 GHz, 73 GHz, and 140 GHz frequency bands for future MU-ultra-mMIMO mmWave and THz wireless communication systems [ 136 , 137 ]. Therefore, system performance is verified at these frequencies using the simulation parameters shown in Tables 2 and 3 for a maximum of 256 × 16 MIMO order with 4 users and 8 users. At the BS, rectangular antennas array is used of size 256 (64 rows and 4 columns) is connected with 4-RF chains and at MS, 16-element (4 rows and 4 columns) square array antenna is used with 4-RF chains. With this design, every antenna element is connected to four phase shifters and radiation patterns of antenna arrays becomes isotropic with linear or rectangular geometry. In order to have peak spectral efficiencies, independent channels are assigned for each user and independent data streams are transmitted through each RF chain that leads to maximum of four data streams per user. The channel between UEs and BS is modeled as well as validated through “scattering-based MIMO spatial channel with single-bounce ray tracing” model. This model assumes the randomly placed scatters and presence of UEs at various T–R spatial locations. The MSs of each user is modeled by compensating thermal noise and path loss. Common channel is used for path loss modelling for both LOS, non-LOS scenarios, data transmissions as well as for channel sounding. The periodic updates of channel matrix at regular intervals are essential to mimic the channel variations.
From Table 4 , it is very clear that the RMS EVM values are minimum when the number of independent data streams per user are high. However, for user 1 (who has four data streams which is maximum), error values are increasing with increasing mMIMO system configurations from 64 × 16 to 256 × 16. When the number of independent data streams per user are minimum (1data stream/user, 2data stream/user), the error values are higher at lower configurations of mMIMO size, however, the RMS EVM values are reducing at higher mMIMO size configurations. From the above simulation results, we can conclude that 256 × 8, 128 × 16, and 64 × 16 mMIMO configurations are optimum for 4-user mMIMO hybrid beamforming systems when the number of independent data streams per user are 2, 4, and 4 respectively.
From Table 5 , the optimum RMS EVM values are achieved for 256 × 16 mMIMO antenna configurations where users maintain 4 independent data streams/user, and error values are highest for 64 × 4 mMIMO configuration with one independent data stream/user. For a given BS antenna arrays and modulation scheme, it is clear that RMS EVM values are decreasing when the users maintain higher number of independent data streams. For the given modulation scheme, the increasing size of BS antenna array leads to decrease in RMS EVM values. However, there are a few cases where there is a little increase RMS EVM values for increasing BS antenna array size. For users 3, 4, and 7 there is little increase in EVM values when the BS antennas are increased from 128 to 256. For user 5 there is a significant increase in EVM values when the BS antennas are increased from 64 to 256. If the user data has divided into multiple number of independent parallel data streams, then it needs less number of active antenna elements to transmit signals. Therefore, it gives trade-off between multiple independent data streams per user and size of T–R antenna arrays.
5.1 Performance of MU-mMIMO Systems for Four Users
Figures 7 and 8 represent the performance in terms of RMS EVM values in a MU-mMIMO hybrid beamforming system for four users at 140 GHz and compared with performance at 28 GHz, 73 GHz (Dilli R). Users 1,2,3, and 4 transmit their data using 4, 1, 2, and 1 number of independent data streams respectively. From the Figs. 7 and 8 , it is observed that RMS EVM values are minimum for users 1 and 3 as they maintain more number of data streams for transmission. Interestingly, for user 4, the error values are lowest at 140 GHz as compared to 28 GHz, and 73 GHz frequencies. It justifies the use of THz frequencies and higher order modulation schemes to achieve higher bandwidths, data rates and spectral efficiencies. For user 3 that maintain two independent data streams, the error values are minimum at 73 GHz and it motivates to study the performance of MU-mMIMO hybrid beamforming at these frequencies. For user 1 which has highest number of data streams, the error values are slightly increasing at 140 GHz compared to 73 GHz, and 28 GHz frequency bands, however the errors are within the acceptable limits. Also for a given order of QAM modulation scheme and carrier frequencies that are considered in this paper, the error values are slightly increasing even at higher order mMIMO antenna array configurations for the users who maintain large number of independent data streams and it needs more explorations to minimize the errors in spite that they are within limits.
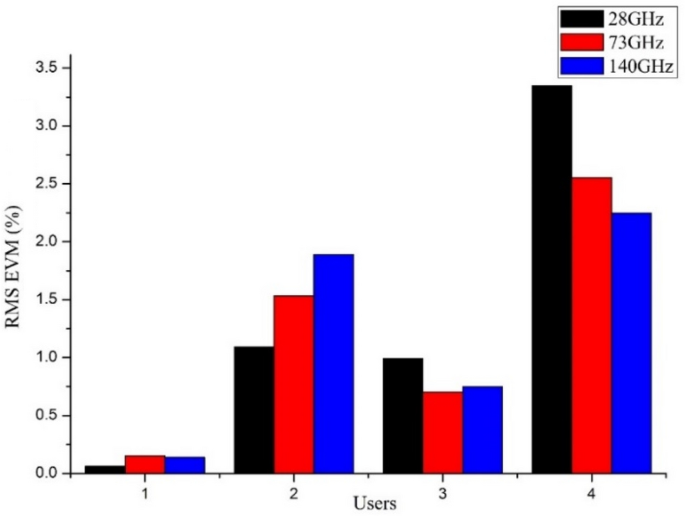
Representation of RMS EVM values using 256-QAM scheme with 64 BS Antennas for four users at 28 GHz, 73 GHz, and 140 GHz frequencies
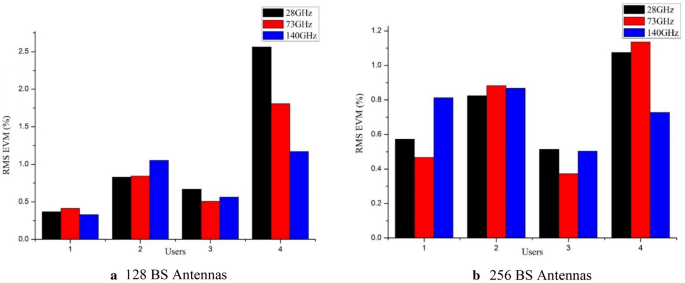
Representation of RMS EVM values using 256-QAM scheme with multiple BS Antennas for four users at 28 GHz, 73 GHz, and 140 GHz frequencies
The signal 3D-radiation patterns in MU-mMIMO hybrid beamforming systems with multiple BS antennas array sizes for 4 users are shown in Fig. 9 . The stronger lobes represent distinct data streams of users and these lobes indicate the spread achieved by hybrid beamforming. From the Fig. 9 , the observation is that radiation beams are becoming more focused for increasing antennas array size at BS and MS that enhances the reliability of signal reception there by throughput.
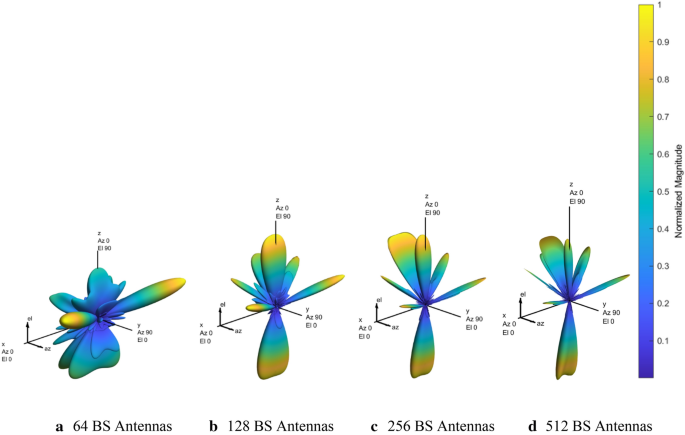
Signal 3D-Radiation pattern of 256-QAM with multiple BS Antennas for four users at 140 GHz
Figures 10 and 11 represent the equalized symbol constellation per data stream with various combinations of QAM modulation schemes and antennas array sizes for 4 users. From the received symbol constellation diagrams of all combinations shown, it is evident that the users with minimum number of independent data streams have higher variance in recovered symbols because of dominant modes are not present in channel and it leads to poor SNR. For the users with multiple data streams, variance of recovered data streams is very minimum as the symbol points are located with minimum dispersion in symbol constellation diagram. Fact is that the data streams use most dominant mode of scattering MIMO channel have maximum SNR values. On the other hand, symbol points in constellation diagrams of users with single data stream are highly dispersed and they have minimum SNR values.
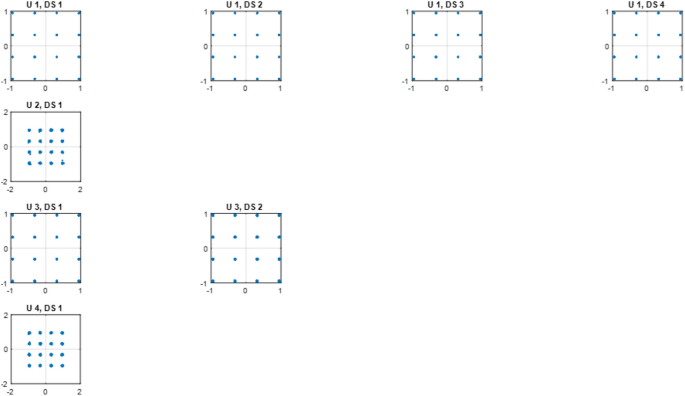
Equalized symbol constellation per data stream of 16-QAM with 64 BS Antennas for four users at 140 GHz
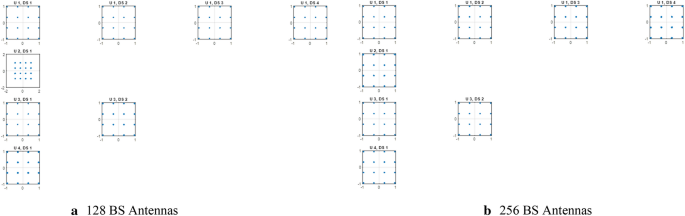
Equalized symbol constellation per data stream of 16-QAM with multiple BS Antennas for four users at 140 GHz
5.2 Performance of MU-mMIMO systems for Eight Users
Figures 12 and 13 represent the performance in terms of RMS EVM values in a MU-mMIMO hybrid beamforming system for eight users at 140 GHz and compared with performance at 28 GHz, 73 GHz (Dilli R et al.,). Users 1,2,3, 4, 5, 6, 7, and 8 transmit their data using 4, 1, 3, 1, 2, 1, 3, and 1 number of independent data streams respectively. From the Figs. 12 and 13 , it is observed that RMS EVM values are minimum for users 1, 3, 5, and 7 as they maintain higher number of data streams for transmission. Interestingly, for user 1, the error values are lowest at all the frequencies as it maintains four independent data streams and the errors are further reduced at 140 GHz as compared to 28 GHz, and 73 GHz frequencies. Users who maintain three or two number of data streams have minimum errors in data transmissions at 140 GHz compared to 28GH, and 73 GHz. It justifies the use of THz frequencies and higher order modulation schemes to achieve higher bandwidths, data rates and spectral efficiencies. User 2 that maintains only one data stream has highest errors in transmission, however the alternate users who have same number of independent data streams leads to lower error values. The common observation is that alternate users with same number of independent data streams undergoes through higher transmission errors. From the overall results at 140 GHz comparing with mmWave frequencies of 28 GHz, and 73 GHz strongly recommends to explore and use the frequencies beyond 100 GHz for next-generation wireless systems.
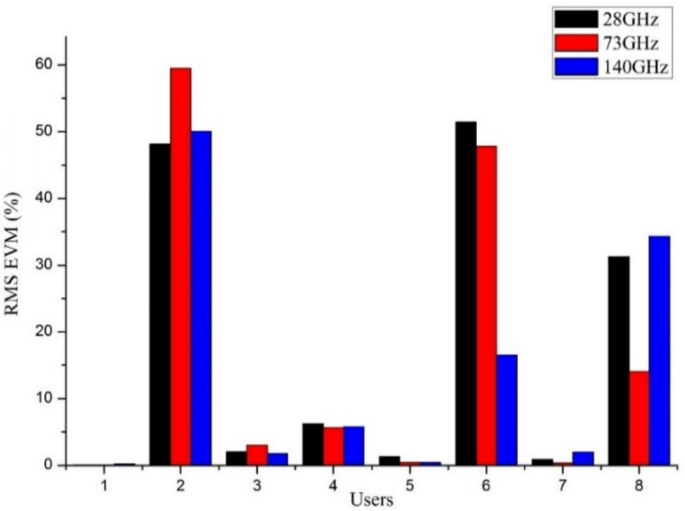
Representation of RMS EVM values using 256-QAM scheme with 64 BS Antennas for eight users
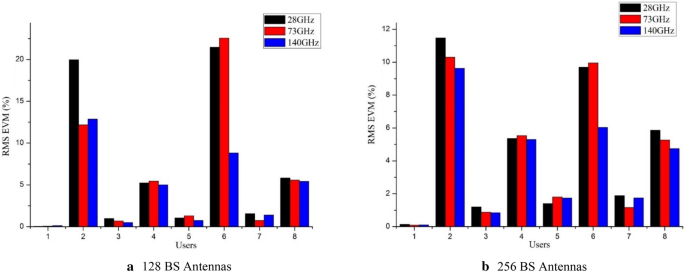
Representation of RMS EVM values using 256-QAM scheme with multiple BS Antennas for eight users
The signal 3D-radiation patterns in MU-mMIMO hybrid beamforming systems with multiple BS antennas array sizes for 8 users are shown in Fig. 14 . The stronger lobes represent distinct data streams of users and these radiation lobes indicate the spread achieved due to hybrid beamforming. From the Fig. 14 , the observation is that radiation beams are becoming more focused for increasing antennas array size at BS and MS which leads to increase in signal reliability there by throughput.
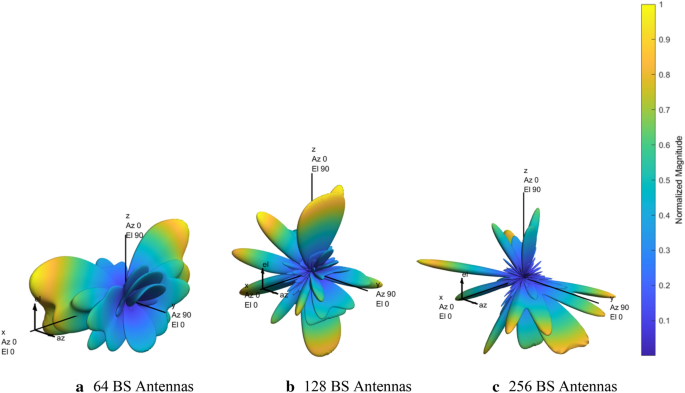
Signal 3D-Radiation pattern of 256-QAM scheme with multiple BS Antennas for eight users at 140 GHz
Figures 15 and 16 represent the equalized symbol constellation per data stream with various combinations of QAM modulation schemes and antennas array sizes for 8 users mMIMO hybrid beamforming system. From the received symbol constellation diagrams of all combinations shown, it is evident that the variance of recovered data streams is minimum for the users with more number of independent data streams as symbol points are located with less dispersion in symbol constellation diagram. Fact is that the data streams use most dominant mode of scattering MIMO channel have maximum SNR values. But, the users with only one independent data stream have highly dispersed received symbols and it is very difficult to estimate the actual transmitted symbols. This higher variance in the received symbols is due to the fact that the absence of dominant modes in channel leads to poor SNR. However, the dispersion is very much reduced with increasing size of antenna arrays at BS and MS as shown in Figs. 15 and 16 . Therefore, it is essential to use higher order antenna arrays to achieve higher spectral efficiency.
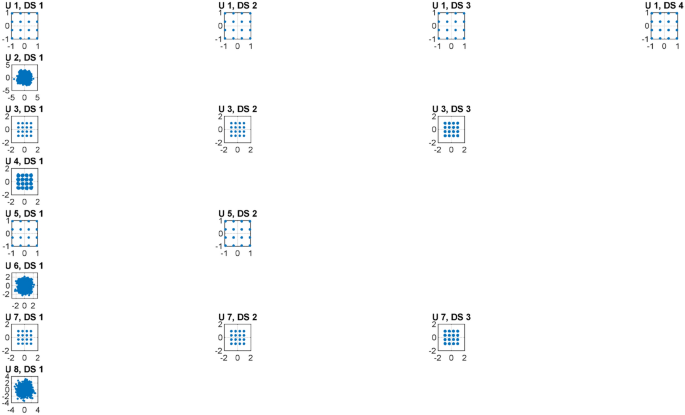
Equalized symbol constellation per data stream of 16-QAM with 64 BS Antennas for eight users at 140 GHz
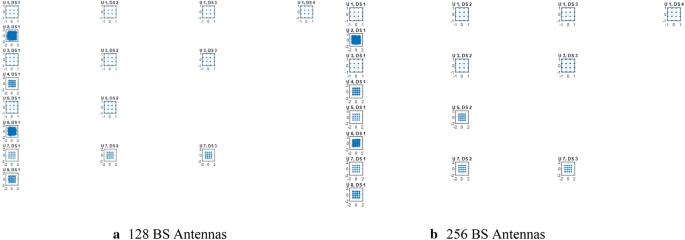
Equalized symbol constellation per data stream of 16-QAM with multiple BS Antennas for eight users at 140 GHz
6 Conclusions and Future scope
In this paper, MU-ultra-mMIMO hybrid beamforming system is designed for THz frequencies with many independent data streams per user. Rigorous analysis is performed with different QAM modulation orders as well as antenna array configurations at Tx and Rx. The results of MU-ultra-mMIMO hybrid beamforming system at THz and mmWave frequencies [ 136 ] are compared to prove the feasibility of using THz frequencies for next-generation wireless communication systems. For a 4-user mMIMO hybrid beamforming systems, it is suggested to use 256 × 8, 128 × 16, and 64 × 16 mMIMO system configurations where the number of independent data streams per user are 2,4, and 4 respectively. At the same time, for 8-user mMIMO hybrid beamforming systems, it is suggested to use 256 × 16 mMIMO antenna array configurations where users maintain four independent data streams/user. In both four-user mMIMO and eight-user mMIMO hybrid beamforming, if the user has only one independent data stream then it is essential to have higher order mMIMO antenna arrays at BS and MS in which case 256 × 4 mMIMO configuration is suitable. From the overall results of research work carried at 140 GHz, the main observation is that for users with less number of independent data streams, their RMS EVM values are higher whereas for users with more number of independent data streams, the RMS EVM values are minimum. Therefore, it is strongly recommended to maintain higher number of independent data streams per user to minimize the RMS EVM values and achieve higher order throughputs. However, hybrid beamforming designs with an objective of minimizing RMS EVM values for users with single (or less) number of independent data streams and achieve higher throughput needs to be carried out as a future research direction. Future work also includes performance analysis of the proposed MU-mMIMO hybrid beamforming system for ultra-mMIMO antenna array configurations of order 1024 × 1024 at 0.2THz, 0.34THz, and 0.4THz frequency bands as the atmospheric absorption loss beyond Frii’s free space path loss of the signal is minimum.
Data Availability
Not applicable.
Code Availability
MATLAB code is available in the supplementary material.
Abbreviations
Three dimensional
3Rd generation partnership project
Fifth generation
Sixth generation
Analog-to-digital converter
Artificial intelligence
Bit error rate
Base station
Complex number
Cyclic prefix
Channel quality information
Channel state information
Device-to-device
Electromagnetic
Error vector magnitude
Frequency division duplex
Fast fourier transform
Global position system
Hybrid beamforming with peak search
Independent and identically distributed
Industrial IoT
Internet of everything
Internet of space
Internet of things
Inter symbol interference
Joint spatial division multiplexing
Light detection and ranging
Line of sight
- Multi–input–multi–output
- Massive MIMO
Minimum mean-square error
Maximal ratio combining
Mobile station
Mean square error
Non-line of sight
Non-orthogonal Multiple Access
Orthogonal frequency division multiplexing
Orthogonal matching pursuit
Quadrature amplitude modulation
Quality of service
Radio frequency
Root mean square
Signal-to-interference-plus-noise ratio
Signal-to-noise ratio
Time division duplex
Time–frequency
Transmit-receive
Transmitter
User equipment
Zero forcing
M. H. Alsharif, A. H. Kelechi, M. A. Albreem, S. A. Chaudhry, M. S. Zia and S. Kim, Sixth Generation (6G) Wireless Networks: Vision, Research Activities, Challenges and Potential Solutions, Symmetry , Vol. 12, No. 4, pp. 676, 2020. https://doi.org/10.3390/sym12040676 .
Article Google Scholar
G. Gui, M. Liu, F. Tang, N. Kato and F. Adachi, 6G: Opening New Horizons for Integration of Comfort, Security, and Intelligence, IEEE Wireless Communications , Vol. 27, No. 5, pp. 126–132, 2020. https://doi.org/10.1109/MWC.001.1900516 .
F. Qamar, M. U. A. Siddiqui, M. N. Hindia, R. Hassan and Q. N. Nguyen, Issues, Challenges, and Research Trends in Spectrum Management: A Comprehensive Overview and New Vision for Designing 6G Networks, Electronics , Vol. 9, No. 9, pp. 1416, 2020. https://doi.org/10.3390/electronics9091416 .
T. S. Rappaport, Y. Xing, O. Kanhere, S. Ju, A. Alkhateeb, G. C. Trichopoulos, A. Madanayake, S. Mandal, “Wireless Communications and Applications Above 100 GHz: Opportunities and Challenges for 6G and Beyond,” in IEEE Access, vol. 7, pp. 78729–78757. doi: https://doi.org/10.1109/ACCESS.2019.2921522 .
V. Petrov, D. Moltchanov and Y. Koucheryavy, "Applicability assessment of terahertz information showers for next-generation wireless networks," 2016 IEEE International Conference on Communications (ICC), Kuala Lumpur, 2016, pp. 1–7, doi: https://doi.org/10.1109/ICC.2016.7511129 .
S. Chinchali et al., “Network Offloading Policies for Cloud Robotics: a Learning-based Approach,” arXiv preprint https://arxiv.org/abs/1902.05703 , Feb. 2019.
M. Mozaffari, A. Taleb Zadeh Kasgari, W. Saad, M. Bennis and M. Debbah, "Beyond 5G with UAVs: Foundations of a 3D Wireless Cellular Network," in IEEE Transactions on Wireless Communications , vol. 18, no. 1, pp. 357–372, Jan. 2019, doi: https://doi.org/10.1109/TWC.2018.2879940 .
J. S. Parker, P. Mickelson, J. Yeak, et al., Exploiting the Terahertz Band for Radio navigation, J Infrared Milli Terahz Waves , Vol. 37, pp. 1021–1042, 2016. https://doi.org/10.1007/s10762-016-0291-8 .
D. Damyanov, et al., High Resolution Lensless Terahertz Imaging and Ranging, IEEE Access , Vol. 7, pp. 147704–147712, 2019. https://doi.org/10.1109/ACCESS.2019.2934582 .
O. Kanhere, S. Ju, Y. Xing, and T. S. Rappaport, “Map Assisted Millimeter Wave Localization for Accurate Position Location,” 2019 IEEE Global Communications Conference (GLOBECOM), Waikoloa, HI, USA, 2019, pp. 1-6, doi: https://doi.org/10.1109/GLOBECOM38437.2019.9013365 .
M. Z. Chowdhury, M. Shahjalal, M. K. Hasan and Y. M. Jang, The Role of Optical Wireless Communication Technologies in 5G/6G and IoT Solutions: Prospects, Directions, and Challenges, Applied Science. , Vol. 9, No. 20, pp. 4367, 2019. https://doi.org/10.3390/app9204367 .
S. C. Tokgoz, S. Althunibat and K. Qaraqe, "A Link-Selection Mechanism for Hybrid FSO-mmWave Systems based on Index Modulation," ICC 2020 - 2020 IEEE International Conference on Communications (ICC) , Dublin, Ireland, 2020, pp. 1–7, doi: https://doi.org/10.1109/ICC40277.2020.9148634 .
A. A. Boulogeorgos, et al., Terahertz Technologies to Deliver Optical Network Quality of Experience in Wireless Systems Beyond 5G, IEEE Communications Magazine , Vol. 56, No. 6, pp. 144–151, 2018. https://doi.org/10.1109/MCOM.2018.1700890 .
R. Dilli “Analysis of 5G Wireless Systems in FR1 and FR2 Frequency Bands" 2020 2nd International Conference on Innovative Mechanisms for Industry Applications (ICIMIA), pp.767–772, Bangalore, India, 2020 doi: https://doi.org/10.1109/ICIMIA48430.2020.9074973 .
I. F. Akyildiz, A. Kak and S. Nie, 6G and Beyond: The Future of Wireless Communications Systems, IEEE Access , Vol. 8, pp. 133995–134030, 2020. https://doi.org/10.1109/ACCESS.2020.3010896 .
M. Z. Chowdhury, M. Shahjalal, S. Ahmed and Y. M. Jang, 6G Wireless Communication Systems: Applications, Requirements, Technologies, Challenges, and Research Directions, IEEE Open Journal of the Communications Society , Vol. 1, pp. 957–975, 2020. https://doi.org/10.1109/OJCOMS.2020.3010270 .
B. Li, Z. Fei and Y. Zhang, UAV Communications for 5G and Beyond: Recent Advances and Future Trends, IEEE Internet of Things Journal , Vol. 6, No. 2, pp. 2241–2263, 2019. https://doi.org/10.1109/JIOT.2018.2887086 .
O. Esrafilian, R. Gangula and D. Gesbert, Learning to Communicate in UAV-Aided Wireless Networks: Map-Based Approaches, IEEE Internet of Things Journal , Vol. 6, No. 2, pp. 1791–1802, 2019. https://doi.org/10.1109/JIOT.2018.2879682 .
Yiqing Zhou, Ling Liu, Lu Wang, Ning Hui, Xinyu Cui, Jie Wu, Yan Peng, Yanli Qi, Chengwen Xing, “Service-aware 6G: An intelligent and open network based on the convergence of communication, computing and caching”, Digital Communications and Networks, Volume 6, Issue 3, 2020, Pages 253–260, ISSN 2352–8648, https://doi.org/10.1016/j.dcan.2020.05.003 .
A. Ahad, M. Tahir, M. Aman Sheikh, K. I. Ahmed, A. Mughees and A. Numani, Technologies Trend towards 5G Network for Smart Health-Care Using IoT: A Review, Sensors , Vol. 20, No. 14, pp. 4047, 2020. https://doi.org/10.3390/s20144047 .
K. David and H. Berndt, 6G Vision and Requirements: Is There Any Need for Beyond 5G?, IEEE Vehicular Technology Magazine , Vol. 13, No. 3, pp. 72–80, 2018. https://doi.org/10.1109/MVT.2018.2848498 .
John F.O’Hara, Sabit Ekin, Wooyeol Choi, Ickhyun Song “A Perspective on Terahertz Next-Generation Wireless Communications” Technologies 2019, 7 (2), 43; https://doi.org/10.3390/technologies7020043
W. Saad, M. Bennis and M. Chen, A Vision of 6G Wireless Systems: Applications, Trends, Technologies, and Open Research Problems, IEEE Network , Vol. 34, No. 3, pp. 134–142, 2020. https://doi.org/10.1109/MNET.001.1900287 .
P. Yang, Y. Xiao, M. Xiao and S. Li, 6G Wireless Communications: Vision and Potential Techniques, IEEE Network , Vol. 33, No. 4, pp. 70–75, 2019. https://doi.org/10.1109/MNET.2019.1800418 .
Article MathSciNet Google Scholar
Kurşat Tekbıyık, Ali Rıza Ekti, Guneş Karabulut Kurt, Ali Gorcin, “Terahertz band communication systems: Challenges, novelties and standardization efforts” Physical Communication, Volume 35, 2019, 100700, ISSN 1874-4907, https://doi.org/10.1016/j.phycom.2019.04.014 .
Yang Lu “Security in 6G: The Prospects and the Relevant Technologies” Journal of Industrial Integration and Management Vol. 05, No. 03, pp. 271–289 (2020), https://doi.org/10.1142/S2424862220500165
F. Ian, Akyildiz, Josep Miquel Jornet, Chong Han, “Terahertz band: Next frontier for wireless communications,” Physical Communication , Vol. 12, pp. 16–32, 2014. https://doi.org/10.1016/j.phycom.2014.01.006 .
D. M. Mittleman, Perspective: Terahertz science and technology, Journal of Applied Physics , Vol. 122, No. 23, 230901, 2017. https://doi.org/10.1063/1.5007683 .
N. Kato, B. Mao, F. Tang, Y. Kawamoto and J. Liu, Ten Challenges in Advancing Machine Learning Technologies toward 6G, IEEE Wireless Communications , Vol. 27, No. 3, pp. 96–103, 2020. https://doi.org/10.1109/MWC.001.1900476 .
K. B. Letaief, W. Chen, Y. Shi, J. Zhang and Y. A. Zhang, The Roadmap to 6G: AI Empowered Wireless Networks, IEEE Communications Magazine , Vol. 57, No. 8, pp. 84–90, 2019. https://doi.org/10.1109/MCOM.2019.1900271 .
F. Tang, Y. Kawamoto, N. Kato and J. Liu, Future Intelligent and Secure Vehicular Network Toward 6G: Machine-Learning Approaches, Proceedings of the IEEE , Vol. 108, No. 2, pp. 292–307, 2020. https://doi.org/10.1109/JPROC.2019.2954595 .
K. Chen, T. Zhang, R. D. Gitlin and G. Fettweis, Ultra-Low Latency Mobile Networking, IEEE Network , Vol. 33, No. 2, pp. 181–187, 2019. https://doi.org/10.1109/MNET.2018.1800011 .
S. Lien, Y. Kuo, D. Deng, H. Tsai, A. Vinel and A. Benslimane, Latency-Optimal mmWave Radio Access for V2X Supporting Next Generation Driving Use Cases, IEEE Access , Vol. 7, pp. 6782–6795, 2019. https://doi.org/10.1109/ACCESS.2018.2888868 .
Yun Chen, Wenfeng Liu, Zhiang Niu, Zhongxiu Feng, Qiwei Hu, Tao Jiang, Pervasive intelligent endogenous 6G wireless systems: Prospects, theories and key technologies, Digital Communications and Networks, Volume 6, Issue 3, 2020, Pages 312–320, ISSN 2352–8648, https://doi.org/10.1016/j.dcan.2020.07.002 .
S. J. Nawaz, S. K. Sharma, S. Wyne, M. N. Patwary and M. Asaduzzaman, Quantum Machine Learning for 6G Communication Networks: State-of-the-Art and Vision for the Future, IEEE Access , Vol. 7, pp. 46317–46350, 2019. https://doi.org/10.1109/ACCESS.2019.2909490 .
X. Shen, et al., AI-Assisted Network-Slicing Based Next-Generation Wireless Networks, IEEE Open Journal of Vehicular Technology , Vol. 1, pp. 45–66, 2020. https://doi.org/10.1109/OJVT.2020.2965100 .
Volker Ziegler, Harish Viswanathan, Hannu Flinck, Marco Hoffmann, Vilho Raisanen, and Kimmo Hatonen “6G Architecture to Connect the Worlds” VOLUME 8, 2020, pg: 173508–173520, IEEE Access, October 1, 2020. DOI: https://doi.org/10.1109/ACCESS.2020.3025032
R. Shafin, L. Liu, V. Chandrasekhar, H. Chen, J. Reed and J. C. Zhang, Artificial Intelligence-Enabled Cellular Networks: A Critical Path to Beyond-5G and 6G, IEEE Wireless Communications , Vol. 27, No. 2, pp. 212–217, 2020. https://doi.org/10.1109/MWC.001.1900323 .
B. Mao, Y. Kawamoto and N. Kato, AI-Based Joint Optimization of QoS and Security for 6G Energy Harvesting Internet of Things, IEEE Internet of Things Journal , Vol. 7, No. 8, pp. 7032–7042, 2020. https://doi.org/10.1109/JIOT.2020.2982417 .
M. S. Sim, Y. Lim, S. H. Park, L. Dai and C. Chae, Deep Learning-Based mmWave Beam Selection for 5G NR/6G with Sub-6GHz Channel Information: Algorithms and Prototype Validation, IEEE Access , Vol. 8, pp. 51634–51646, 2020. https://doi.org/10.1109/ACCESS.2020.2980285 .
Y. Zhang, B. Di, P. Wang, J. Lin and L. Song, HetMEC: Heterogeneous Multi-Layer Mobile Edge Computing in the 6G Era, IEEE Transactions on Vehicular Technology , Vol. 69, No. 4, pp. 4388–4400, 2020. https://doi.org/10.1109/TVT.2020.2975559 .
T. Huang, W. Yang, J. Wu, J. Ma, X. Zhang and D. Zhang, A Survey on Green 6G Network: Architecture and Technologies, IEEE Access , Vol. 7, pp. 175758–175768, 2019. https://doi.org/10.1109/ACCESS.2019.2957648 .
N. Kato, et al., Optimizing Space-Air-Ground Integrated Networks by Artificial Intelligence, IEEE Wireless Communications , Vol. 26, No. 4, pp. 140–147, 2019. https://doi.org/10.1109/MWC.2018.1800365 .
T. Lin and Y. Zhu, Beamforming Design for Large-Scale Antenna Arrays Using Deep Learning, IEEE Wireless Communications Letters , Vol. 9, No. 1, pp. 103–107, 2020. https://doi.org/10.1109/LWC.2019.2943466 .
Jing JIANG, Yue LI, Long CHEN, Jianbo DU & Chunguo LI “Multitask deep learning-based multiuser hybrid beamforming for mm-wave orthogonal frequency division multiple access systems”. SCIENCE CHINA Information Sciences August 2020, Vol. 63 180303:1–180303:11 https://doi.org/10.1007/s11432-020-2937-y
inh C. Nguyen, Pubudu N. Pathirana, Ming Ding, Aruna Seneviratne, Blockchain for 5G and beyond networks: A state of the art survey, Journal of Network and Computer Applications, Volume 166, 2020, 102693, ISSN 1084-8045, https://doi.org/10.1016/j.jnca.2020.102693
T. Nguyen, N. Tran, L. Loven, J. Partala, M. Kechadi and S. Pirttikangas, "Privacy-Aware Blockchain Innovation for 6G: Challenges and Opportunities ," 2020 2nd 6G Wireless Summit (6G SUMMIT) , Levi, Finland, 2020, pp. 1-5, doi: https://doi.org/10.1109/6GSUMMIT49458.2020.9083832 .
S. Dang, O. Amin, B. Shihada, et al., What should 6G be?, Nat Electron , Vol. 3, pp. 20–29, 2020. https://doi.org/10.1038/s41928-019-0355-6 .
J. Ma, R. Shrestha, J. Adelberg, et al., Security and eavesdropping in terahertz wireless links, Nature , Vol. 563, pp. 89–93, 2018. https://doi.org/10.1038/s41586-018-0609-x .
J. Xu, W. Xu, D. W. K. Ng and A. L. Swindle hurst, "Secure Communication for Spatially Sparse Millimeter-Wave Massive MIMO Channels via Hybrid Precoding," in IEEE Transactions on Communications, vol. 68, no. 2, pp. 887–901, Feb. 2020, doi: https://doi.org/10.1109/TCOMM.2019.2954517 .
V. Petrov, J. M. Eckhardt, D. Moltchanov, Y. Koucheryavy and T. Kurner, "Measurements of Reflection and Penetration Losses in Low Terahertz Band Vehicular Communications," 2020 14th European Conference on Antennas and Propagation (EuCAP), Copenhagen, Denmark, 2020, pp. 1–5, doi: https://doi.org/10.23919/EuCAP48036.2020.9135389 .
G. R. MacCartney, S. Deng, S. Sun and T. S. Rappaport, "Millimeter-Wave Human Blockage at 73GHz with a Simple Double Knife-Edge Diffraction Model and Extension for Directional Antennas," 2016 IEEE 84th Vehicular Technology Conference (VTC-Fall), Montreal, QC, 2016, pp. 1-6, doi: https://doi.org/10.1109/VTCFall.2016.7881087 .
S. Ju et al., "Scattering Mechanisms and Modeling for Terahertz Wireless Communications," ICC 2019 - 2019 IEEE International Conference on Communications (ICC), Shanghai, China, 2019, pp. 1–7, doi: https://doi.org/10.1109/ICC.2019.8761205 .
A. A. Goulianos et al., "Measurements and Characterisation of Surface Scattering at 60 GHz," 2017 IEEE 86th Vehicular Technology Conference (VTC-Fall), Toronto, ON, 2017, pp. 1-5, doi: https://doi.org/10.1109/VTCFall.2017.8288373 .
L. Pometcu and R. D'Errico, "Characterization of sub-THz and mmwave propagation channel for indoor scenarios," 12th European Conference on Antennas and Propagation (EuCAP 2018), London, 2018, pp. 1–4, doi: https://doi.org/10.1049/cp.2018.0991 .
ITU-R, “Specific attenuation model for rain for use in prediction methods, propagation in non-ionized media,” Tech. Rep. P.838–3, Mar. 2005.
J. Ma, J. Adelberg, R. Shrestha, et al., The Effect of Snow on a Terahertz Wireless Data Link, J Infrared Milli Terahz Waves , Vol. 39, pp. 505–508, 2018. https://doi.org/10.1007/s10762-018-0486-2 .
ITU-R, “Attenuation by Atmospheric Gases and related effects” Tech. Rep. P.676–12, Aug. 2019.
Jh. Zhang, P. Tang, L. Yu, et al., Channel measurements and models for 6G: current status and future outlook, Front Inform Technol Electron Eng , Vol. 21, pp. 39–61, 2020. https://doi.org/10.1631/FITEE.1900450 .
G. R. MacCartney and T. S. Rappaport, A Flexible Millimeter-Wave Channel Sounder With Absolute Timing, IEEE Journal on Selected Areas in Communications , Vol. 35, No. 6, pp. 1402–1418, 2017. https://doi.org/10.1109/JSAC.2017.2687838 .
S. Priebe, C. Jastrow, M. Jacob, T. Kleine-Ostmann, T. Schrader and T. Kürner, Channel and Propagation Measurements at 300GHz, IEEE Transactions on Antennas and Propagation , Vol. 59, No. 5, pp. 1688–1698, 2011. https://doi.org/10.1109/TAP.2011.2122294 .
Shihao Ju, Ojas Kanhere, Yunchou Xing, and Theodore S. Rappaport. 2019. A Millimeter-Wave Channel Simulator NYUSIM with Spatial Consistency and Human Blockage. In 2019 IEEE Global Communications Conference (GLOBECOM). IEEE Press, 1–6. DOI: https://doi.org/10.1109/GLOBECOM38437.2019.9013273
3GPP, “Technical Specification Group Radio Access Network; Study on channel model for frequencies from 0.5 to 100 GHz (Release 14),” TR 38.901 V14.2.0, Sept. 2017. [Online]. Available: http://www.3gpp.org/DynaReport/38901.htm
3GPP, “Technical Specification Group Radio Access Network; Study on Scenarios and Requirements for Next Generation Access Technologies (Release 15),” 3rd Generation Partnership Project (3GPP), TR 38.901 V15.0.0, June 2018.
3GPP, “Study on channel model for frequencies from 0.5 to 100 GHz,” TR 38.901 V14.0.0, May 2017.
Aalto University, AT&T, BUPT, CMCC, Ericsson, Huawei, Intel, KT Corporation, Nokia, NTT DOCOMO, New York University, Qualcomm, Samsung, University of Bristol, and University of Southern California, “5G Channel Model for bands up to 100 GHz,” Tech. Rep., Oct. 2016. [Online]. Available: http://www.5gworkshops.com/5GCM.html
S. Sun, T. S. Rappaport, M. Shafi, P. Tang, J. Zhang and P. J. Smith, Propagation Models and Performance Evaluation for 5G Millimeter-Wave Bands, IEEE Transactions on Vehicular Technology , Vol. 67, No. 9, pp. 8422–8439, 2018. https://doi.org/10.1109/TVT.2018.2848208 .
J. F. Harvey, M. B. Steer and T. S. Rappaport, Exploiting High Millimeter Wave Bands for Military Communications, Applications, and Design, IEEE Access , Vol. 7, pp. 52350–52359, 2019. https://doi.org/10.1109/ACCESS.2019.2911675 .
T. S. Rappaport, Y. Xing, G. R. MacCartney, A. F. Molisch, E. Mellios and J. Zhang, Overview of Millimeter Wave Communications for Fifth-Generation (5G) Wireless Networks—With a Focus on Propagation Models, IEEE Transactions on Antennas and Propagation , Vol. 65, No. 12, pp. 6213–6230, 2017. https://doi.org/10.1109/TAP.2017.2734243 .
Manoj A and A. P. Kannu, "Channel Estimation Strategies for Multi-User mmWave Systems," in IEEE Transactions on Communications, vol. 66, no. 11, pp. 5678–5690, Nov. 2018, doi: https://doi.org/10.1109/TCOMM.2018.2854188 .
X. Ge, Y. Sun, H. Gharavi and J. Thompson, Joint Optimization of Computation and Communication Power in Multi-User Massive MIMO Systems, IEEE Transactions on Wireless Communications , Vol. 17, No. 6, pp. 4051–4063, 2018. https://doi.org/10.1109/TWC.2018.2819653 .
A. N. Uwaechia, N. M. Mahyuddin, M. F. Ain, N. M. Abdul Latiff and N. F. Za'bah, "Multi-user mmWave MIMO Channel Estimation with Hybrid Beamforming over Frequency Selective Fading Channels," 2019 IEEE International Conference on Signal and Image Processing Applications (ICSIPA), Kuala Lumpur, Malaysia, 2019, pp. 46–51, doi: https://doi.org/10.1109/ICSIPA45851.2019.8977776 .
X. Rao and V. K. N. Lau, "Distributed Compressive CSIT Estimation and Feedback for FDD Multi-User Massive MIMO Systems," in IEEE Transactions on Signal Processing, vol. 62, no. 12, pp. 3261–3271, June15, 2014, doi: https://doi.org/10.1109/TSP.2014.2324991 .
K. Guan, et al., On Millimeter Wave and THz Mobile Radio Channel for Smart Rail Mobility, IEEE Transactions on Vehicular Technology , Vol. 66, No. 7, pp. 5658–5674, 2017. https://doi.org/10.1109/TVT.2016.2624504 .
K. Guan et al., "Channel Sounding and Ray Tracing for Intra-Wagon Scenario at mmWave and Sub-mmWave Bands," in IEEE Transactions on Antennas and Propagation, doi: https://doi.org/10.1109/TAP.2020.3016399 .
S. Bhardwaj, N. K. Nahar and J. L. Volakis, All electronic propagation loss measurement and link budget analysis for 350 GHz communication link, Microw. Opt. Technol. Lett. , Vol. 59, pp. 415–423, 2017. https://doi.org/10.1002/mop.30307 .
Y. Xing and T. S. Rappaport, "Propagation Measurement System and Approach at 140 GHz-Moving to 6G and Above 100 GHz," 2018 IEEE Global Communications Conference (GLOBECOM), Abu Dhabi, United Arab Emirates, 2018, pp. 1-6, doi: https://doi.org/10.1109/GLOCOM.2018.8647921 .
S. Kim, W. T. Khan, A. Zajić and J. Papapolymerou, D-Band Channel Measurements and Characterization for Indoor Applications, IEEE Transactions on Antennas and Propagation , Vol. 63, No. 7, pp. 3198–3207, 2015. https://doi.org/10.1109/TAP.2015.2426831 .
Article MathSciNet MATH Google Scholar
G. R. Maccartney, T. S. Rappaport, S. Sun and S. Deng, Indoor Office Wideband Millimeter-Wave Propagation Measurements and Channel Models at 28 and 73 GHz for Ultra-Dense 5G Wireless Networks, IEEE Access , Vol. 3, pp. 2388–2424, 2015. https://doi.org/10.1109/ACCESS.2015.2486778 .
G. R. Mac Cartney and T. S. Rappaport, "Rural Macrocell Path Loss Models for Millimeter Wave Wireless Communications," in IEEE Journal on Selected Areas in Communications, vol. 35, no. 7, pp. 1663–1677, July 2017, doi: https://doi.org/10.1109/JSAC.2017.2699359 .
Q. Xia and J. M. Jornet, Expedited Neighbor Discovery in Directional Terahertz Communication Networks Enhanced by Antenna Side-Lobe Information, IEEE Transactions on Vehicular Technology , Vol. 68, No. 8, pp. 7804–7814, 2019. https://doi.org/10.1109/TVT.2019.2924820 .
Yunchou Xing, Ojas Kanhere, Shihao Ju, and Theodore S. Rappaport. 2019. Indoor Wireless Channel Properties at Millimeter Wave and Sub-Terahertz Frequencies. In 2019 IEEE Global Communications Conference (GLOBECOM). IEEE Press, 1–6. DOI: https://doi.org/10.1109/GLOBECOM38437.2019.9013236
M. M. Abdulwahid, O. A. S. Al-Ani, M. F. Mosleh and R. A. Abd-Alhameed, "Investigation of Millimeter-Wave Indoor Propagation at Different Frequencies," 2019 4th Scientific International Conference Najaf (SICN), Al-Najef, Iraq, 2019, pp. 25-30, doi: https://doi.org/10.1109/SICN47020.2019.9019358 .
G. R. MacCartney and T. S. Rappaport, Millimeter-Wave Base Station Diversity for 5G Coordinated Multipoint (CoMP) Applications, IEEE Transactions on Wireless Communications , Vol. 18, No. 7, pp. 3395–3410, 2019. https://doi.org/10.1109/TWC.2019.2913414 .
M. Khatun, C. Guo, D. Matolak and H. Mehrpouyan, "Indoor and Outdoor Penetration Loss Measurements at 73 and 81GHz," 2019 IEEE Global Communications Conference (GLOBECOM), Waikoloa, HI, USA, 2019, pp. 1-5, doi: https://doi.org/10.1109/GLOBECOM38437.2019.9013945 .
Y. Xing, O. Kanhere, S. Ju, T. S. Rappaport and G. R. MacCartney, "Verification and Calibration of Antenna Cross-Polarization Discrimination and Penetration Loss for Millimeter Wave Communications," 2018 IEEE 88th Vehicular Technology Conference (VTC-Fall), Chicago, IL, USA, 2018, pp. 1-6, doi: https://doi.org/10.1109/VTCFall.2018.8690683 .
T. S. Rappaport and S. Deng, "73 GHz wideband millimeter-wave foliage and ground reflection measurements and models," 2015 IEEE International Conference on Communication Workshop (ICCW), London, 2015, pp. 1238–1243, doi: https://doi.org/10.1109/ICCW.2015.7247347 .
J. Ryan, G. R. MacCartney and T. S. Rappaport, "Indoor office wideband penetration loss measurements at 73 GHz," 2017 IEEE International Conference on Communications Workshops (ICC Workshops), Paris, 2017, pp. 228–233, doi: https://doi.org/10.1109/ICCW.2017.7962662 .
G. R. MacCartney and T. S. Rappaport, "73GHz millimeter wave propagation measurements for outdoor urban mobile and backhaul communications in New York City," 2014 IEEE International Conference on Communications (ICC), Sydney, NSW, 2014, pp. 4862–4867, doi: https://doi.org/10.1109/ICC.2014.6884090 .
G. R. MacCartney, S. Deng and T. S. Rappaport, "Indoor Office Plan Environment and Layout-Based mmWave Path Loss Models for 28 GHz and 73 GHz," 2016 IEEE 83rd Vehicular Technology Conference (VTC Spring), Nanjing, 2016, pp. 1-6, doi: https://doi.org/10.1109/VTCSpring.2016.7504287 .
J. Ma, R. Shrestha, L. Moeller and D. M. Mittleman, Channel performance for indoor and outdoor terahertz wireless links, APL Photonics , Vol. 3, 051601, 2018. https://doi.org/10.1063/1.5014037 .
J. Kokkoniemi, J. Lehtomaki and M. Juntti, "Measurements on penetration loss in terahertz band," 2016 10th European Conference on Antennas and Propagation (EuCAP), Davos, 2016, pp. 1–5, doi: https://doi.org/10.1109/EuCAP.2016.7481176 .
Chataut, Robin, and Robert Akl. “Massive MIMO Systems for 5G and Beyond Networks-Overview, Recent Trends, Challenges, and Future Research Direction.” Sensors (Basel, Switzerland) vol. 20,10 2753. 12 May. 2020, doi: https://doi.org/10.3390/s20102753
M. Giordani, M. Polese, A. Roy, D. Castor and M. Zorzi, "A Tutorial on Beam Management for 3GPP NR at mmWave Frequencies," in IEEE Communications Surveys & Tutorials , vol. 21, no. 1, pp. 173–196, First quarter 2019, doi: https://doi.org/10.1109/COMST.2018.2869411 .
Silva MMd, Guerreiro J. “On the 5G and Beyond”. Applied Sciences. 2020; 10(20):7091 https://doi.org/10.3390/app10207091
By. Duan, Evolution and innovation of antenna systems for beyond 5G and 6G, Frontiers of Information Technology & Electronic Engineering , Vol. 21, pp. 1–3, 2020. https://doi.org/10.1631/FITEE.2010000 .
Ian F. Akyildiz, Josep Miquel Jornet, Realizing Ultra-Massive MIMO (1024×1024) communication in the (0.06–10) Terahertz band, Nano Communication Networks, Volume 8, 2016, Pages 46–54, ISSN 1878–7789, https://doi.org/10.1016/j.nancom.2016.02.001
H. Gao, Y. Su, S. Zhang and M. Diao, Antenna selection and power allocation design for 5G massive MIMO uplink networks, China Communications , Vol. 16, No. 4, pp. 1–15, 2019.
Google Scholar
M. Giordani, M. Polese, A. Roy, D. Castor and M. Zorzi, Standalone and Non-Standalone Beam Management for 3GPP NR at mmWaves, IEEE Communications Magazine , Vol. 57, No. 4, pp. 123–129, 2019. https://doi.org/10.1109/MCOM.2019.1800384 .
G. Zang, Y. Cui, H. V. Cheng, F. Yang, L. Ding and H. Liu, Optimal Hybrid Beamforming for Multiuser Massive MIMO Systems with Individual SINR Constraints, IEEE Wireless Communications Letters , Vol. 8, No. 2, pp. 532–535, 2019. https://doi.org/10.1109/LWC.2018.2878766 .
J. Li, L. Xiao, X. Xu and S. Zhou, Robust and Low Complexity Hybrid Beamforming for Uplink Multiuser MmWave MIMO Systems, IEEE Communications Letters , Vol. 20, No. 6, pp. 1140–1143, 2016. https://doi.org/10.1109/LCOMM.2016.2542161 .
Vishnu V. Ratnam, Andreas F. Molisch, Ozgun Y. Bursalioglu, and Haralabos C. Papadopoulos. 2018. Hybrid Beamforming with Selection for Multiuser Massive MIMO Systems. IEEE Transactions on Signal Processing, Vol. 66, No. 15, August 1, 2018, 4105–4120. https://doi.org/10.1109/TSP.2018.2838557
Jiang Jing, Kong Deting, “Joint User Scheduling and MU-MIMO Hybrid Beamforming Algorithm for mmWave FDMA Massive MIMO System” International Journal of Antennas and Propagation Volume 2016, Article ID 4341068, 10 pages http://dx.doi.org/ https://doi.org/10.1155/2016/4341068
J. Deng, O. Tirkkonen and C. Studer, "MmWave channel estimation via atomic norm minimization for multi-user hybrid precoding," 2018 IEEE Wireless Communications and Networking Conference (WCNC), Barcelona, 2018, pp. 1-6, doi: https://doi.org/10.1109/WCNC.2018.8377093 .
T. E. Bogale and L. B. Le, "Beamforming for multiuser massive MIMO systems: Digital versus hybrid analog-digital," 2014 IEEE Global Communications Conference, Austin, TX, 2014, pp. 4066-4071, doi: https://doi.org/10.1109/GLOCOM.2014.7037444 .
Lin, T., Cong, J., Zhu, Y., Zhang, J., & Letaief, K. B. (2019). Hybrid Beamforming for Millimeter Wave Systems Using the MMSE Criterion. IEEE Transactions on Communications , 67 (5), 3693–3708. [8616797]. https://doi.org/10.1109/TCOMM.2019.2893632
J. Du, W. Xu, C. Zhao and L. Vandendorpe, "Hybrid Beamforming Design for Multiuser Massive MIMO-OFDM Systems," 2018 15th International Symposium on Wireless Communication Systems (ISWCS), Lisbon, 2018, pp. 1-6, doi: https://doi.org/10.1109/ISWCS.2018.8491217 .
N. Song, T. Yang and H. Sun, "Efficient Hybrid Beamforming for Relay Assisted Millimeter-Wave Multi-User Massive MIMO," 2019 IEEE Wireless Communications and Networking Conference (WCNC) , Marrakesh, Morocco, 2019, pp. 1-6, doi: https://doi.org/10.1109/WCNC.2019.8886016 .
Saeed Ali Saeed Alquhaif, Ishtiaq Ahmad, Mahnoor Rasheed, Ali Raza “An Optimized Hybrid Beamforming for Millimeter Wave MU-Massive MIMO System” DOI: http://dx.doi.org/ https://doi.org/10.17993/3ctecno.2019.specialissue.09
H. Q. Ngo, E. G. Larsson and T. L. Marzetta, Energy and Spectral Efficiency of Very Large Multiuser MIMO Systems, IEEE Transactions on Communications , Vol. 61, No. 4, pp. 1436–1449, 2013. https://doi.org/10.1109/TCOMM.2013.020413.110848 .
Y. Zhao, I. G. Niemegeers and S. H. De Groot, "Distributed mmWave Massive MIMO: A Performance Comparison with a Centralized Architecture for Various Degrees of Hybridization ," 2020 IEEE 8th International Conference on Information, Communication and Networks (ICICN) , Xi'an, China, 2020, pp. 105–110, doi: https://doi.org/10.1109/ICICN51133.2020.9205088 .
X. Wei, C. Hu and L. Dai, "Deep Learning for Beamspace Channel Estimation in Millimeter-Wave Massive MIMO Systems," in IEEE Transactions on Communications , doi: https://doi.org/10.1109/TCOMM.2020.3027027 .
Molisch, A. F., Ratnam, V. V., Han, S., Li, Z., Nguyen, S. L. H., Li, L., & Haneda, K. (2017). Hybrid Beamforming for Massive MIMO: A Survey. IEEE Communications Magazine , 55 ( 9), 134–141. [8030501]. https://doi.org/10.1109/MCOM.2017.1600400
errapragada AK, Kelley B. On the Application of K-User MIMO for 6G Enhanced Mobile Broadband. Sensors (Basel). 2020 Nov 2;20(21):6252. doi: https://doi.org/10.3390/s20216252 .
S. Sun, T. S. Rappaport and M. Shaft, "Hybrid beamforming for 5G millimeter-wave multi-cell networks," IEEE INFOCOM 2018 - IEEE Conference on Computer Communications Workshops (INFOCOM WKSHPS), Honolulu, HI, 2018, pp. 589–596, doi: https://doi.org/10.1109/INFCOMW.2018.8406871 .
J. Du, J. Li, J. He, Y. Guan and H. Lin, Low-Complexity Joint Channel Estimation for Multi-User mmWave Massive MIMO Systems, Electronics , Vol. 9, No. 2, pp. 301, 2020. https://doi.org/10.3390/electronics9020301 .
H. Lin, F. Gao, S. Jin and G. Y. Li, A New View of Multi-User Hybrid Massive MIMO: Non-Orthogonal Angle Division Multiple Access, IEEE Journal on Selected Areas in Communications , Vol. 35, No. 10, pp. 2268–2280, 2017. https://doi.org/10.1109/JSAC.2017.2725682 .
Y. Zeng, L. Yang and R. Zhang, Multi-User Millimeter Wave MIMO with Full-Dimensional Lens Antenna Array, IEEE Transactions on Wireless Communications , Vol. 17, No. 4, pp. 2800–2814, 2018. https://doi.org/10.1109/TWC.2018.2803180 .
Y. Zhang, J. Du, Y. Chen, M. Han and X. Li, Optimal Hybrid Beamforming Design for Millimeter-Wave Massive Multi-User MIMO Relay Systems, IEEE Access , Vol. 7, pp. 157212–157225, 2019. https://doi.org/10.1109/ACCESS.2019.2949786 .
X. Xue, T. E. Bogale, X. Wang, Y. Wang and B. L. Long, "Hybrid analog-digital beamforming for multiuser MIMO millimeter wave relay systems," 2015 IEEE/CIC International Conference on Communications in China (ICCC), Shenzhen, 2015, pp. 1–7, doi: https://doi.org/10.1109/ICCChina.2015.7448664 .
Q. Qin, L. Gui, P. Cheng and B. Gong, Time-Varying Channel Estimation for Millimeter Wave Multiuser MIMO Systems, IEEE Transactions on Vehicular Technology , Vol. 67, No. 10, pp. 9435–9448, 2018. https://doi.org/10.1109/TVT.2018.2854735 .
X. Wu, D. Liu and F. Yin, "Hybrid Beamforming for Multi-User Massive MIMO Systems," in IEEE Transactions on Communications, vol. 66, no. 9, pp. 3879–3891, September. 2018, DOI: https://doi.org/10.1109/TCOMM.2018.2829511 .
Jing Jiang, Ming Lei, and Huanhuan Hou “Downlink Multiuser Hybrid Beamforming for MmWave Massive MIMO-NOMA System with Imperfect CSI” International Journal of Antennas and Propagation Volume 2019, Article ID 9764958, 10 pages https://doi.org/10.1155/2019/9764958
L. Chen and X. Yuan, Blind Multiuser Detection in Massive MIMO Channels with Clustered Sparsity, IEEE Wireless Communications Letters , Vol. 8, No. 4, pp. 1052–1055, 2019. https://doi.org/10.1109/LWC.2019.2906168 .
J. P. Gonzalez-Coma, J. Rodriguez-Fernandez, N. Gonzalez-Prelcic, L. Castedo and R. W. Heath, Channel Estimation and Hybrid Precoding for Frequency Selective Multiuser mmWave MIMO Systems, IEEE Journal of Selected Topics in Signal Processing , Vol. 12, No. 2, pp. 353–367, 2018. https://doi.org/10.1109/JSTSP.2018.2819130 .
Q. Shi and M. Hong, Spectral Efficiency Optimization for Millimeter Wave Multiuser MIMO Systems, IEEE Journal of Selected Topics in Signal Processing , Vol. 12, No. 3, pp. 455–468, 2018. https://doi.org/10.1109/JSTSP.2018.2824246 .
N. Song, H. Sun and T. Yang, "Coordinated Hybrid Beamforming for Millimeter Wave Multi-User Massive MIMO Systems," 2016 IEEE Global Communications Conference (GLOBECOM), Washington, DC, 2016, pp. 1-6, doi: https://doi.org/10.1109/GLOCOM.2016.7841700 .
Z. Li, S. Han, and A. F. Molisch. "Hybrid Beamforming Design for Millimeter-Wave Multi-User Massive MIMO Downlink." 2016 IEEE International Conference on Communications (ICC), Kuala Lumpur, 2016, pp. 1–6, DOI: https://doi.org/10.1109/ICC.2016.7510845
O. E. Ayach, S. Rajagopal, S. Abu-Surra, Z. Pi and R. W. Heath, Spatially Sparse Precoding in Millimeter Wave MIMO Systems, IEEE Transactions on Wireless Communications , Vol. 13, No. 3, pp. 1499–1513, 2014. https://doi.org/10.1109/TWC.2014.011714.130846 .
Y. Feng and S. C. Kim, Hybrid precoding based on matrix-adaptive method for multiuser large-scale antenna arrays, PLoS One. , Vol. 12, No. 12, e0188723, 2017. https://doi.org/10.1371/journal.pone.0188723 .
F. Khalid, Hybrid Beamforming for Millimeter Wave Massive Multiuser MIMO Systems Using Regularized Channel Diagonalization, IEEE Wireless Communications Letters , Vol. 8, No. 3, pp. 705–708, 2019. https://doi.org/10.1109/LWC.2018.2886882 .
L. Liang, W. Xu and X. Dong, Low-Complexity Hybrid Precoding in Massive Multiuser MIMO Systems, IEEE Wireless Communications Letters , Vol. 3, No. 6, pp. 653–656, 2014. https://doi.org/10.1109/LWC.2014.2363831 .
Z. Zheng and H. Gharavi, “Spectral and Energy Efficiencies of Millimeter Wave MIMO with Configurable Hybrid Precoding,” IEEE Transactions on Vehicular Technology , 2019. https://doi.org/10.1109/TVT.2019.2909829 .
W. Liu, X. Hou, Y. Kishiyama, L. Chen and T. Asai, Transform Domain Precoding (TDP) for 5G Evolution and 6G, IEEE Signal Processing Letters , Vol. 27, pp. 1145–1149, 2020. https://doi.org/10.1109/LSP.2020.3004071 .
X. Yu, J. Zhang and K. B. Letaief, A Hardware-Efficient Analog Network Structure for Hybrid Precoding in Millimeter Wave Systems, IEEE Journal of Selected Topics in Signal Processing , Vol. 12, No. 2, pp. 282–297, 2018. https://doi.org/10.1109/JSTSP.2018.2814009 .
R. Dilli, Performance analysis of multi user massive MIMO hybrid beamforming systems at millimeter wave frequency bands, Wireless Networks , 2021. https://doi.org/10.1007/s11276-021-02546-w .
S. Sun, T. S. Rappaport, R. W. Heath, A. Nix and S. Rangan, MIMO for millimeter-wave wireless communications: beamforming, spatial multiplexing, or both?, IEEE Communications Magazine , Vol. 52, No. 12, pp. 110–121, 2014. https://doi.org/10.1109/MCOM.2014.6979962 .
Download references
Open access funding provided by Manipal Academy of Higher Education, Manipal. This research received no external funding.
Author information
Authors and affiliations.
Department of Electronics & Communication Engineering, Manipal Institute of Technology, Manipal Academy of Higher Education, Manipal, Udupi District, Karnataka, India
You can also search for this author in PubMed Google Scholar
Contributions
Author has reviewed the latest research on 6G at THz frequencies, designed a novel algorithm for 6G system and verified its performance.
Corresponding author
Correspondence to Ravilla Dilli .
Ethics declarations
Conflict of interest.
The authors declare no conflict of interest.
Ethical Approval
Consent to participate, consent for publication, additional information, publisher's note.
Springer Nature remains neutral with regard to jurisdictional claims in published maps and institutional affiliations.
Supplementary Information
Below is the link to the electronic supplementary material.
Supplementary file1 (DOCX 22 KB)
Rights and permissions.
Open Access This article is licensed under a Creative Commons Attribution 4.0 International License, which permits use, sharing, adaptation, distribution and reproduction in any medium or format, as long as you give appropriate credit to the original author(s) and the source, provide a link to the Creative Commons licence, and indicate if changes were made. The images or other third party material in this article are included in the article's Creative Commons licence, unless indicated otherwise in a credit line to the material. If material is not included in the article's Creative Commons licence and your intended use is not permitted by statutory regulation or exceeds the permitted use, you will need to obtain permission directly from the copyright holder. To view a copy of this licence, visit http://creativecommons.org/licenses/by/4.0/ .
Reprints and permissions
About this article
Dilli, R. Design and Feasibility Verification of 6G Wireless Communication Systems with State of the Art Technologies. Int J Wireless Inf Networks 29 , 93–117 (2022). https://doi.org/10.1007/s10776-021-00546-3
Download citation
Received : 17 May 2021
Revised : 01 September 2021
Accepted : 11 December 2021
Published : 28 December 2021
Issue Date : March 2022
DOI : https://doi.org/10.1007/s10776-021-00546-3
Share this article
Anyone you share the following link with will be able to read this content:
Sorry, a shareable link is not currently available for this article.
Provided by the Springer Nature SharedIt content-sharing initiative
- Next-generation wireless networks
- THz frequency bands
- Hybrid beamforming
- Find a journal
- Publish with us
- Track your research
Research and development for practical use of underwater optical wireless communication
Ieee account.
- Change Username/Password
- Update Address
Purchase Details
- Payment Options
- Order History
- View Purchased Documents
Profile Information
- Communications Preferences
- Profession and Education
- Technical Interests
- US & Canada: +1 800 678 4333
- Worldwide: +1 732 981 0060
- Contact & Support
- About IEEE Xplore
- Accessibility
- Terms of Use
- Nondiscrimination Policy
- Privacy & Opting Out of Cookies
A not-for-profit organization, IEEE is the world's largest technical professional organization dedicated to advancing technology for the benefit of humanity. © Copyright 2024 IEEE - All rights reserved. Use of this web site signifies your agreement to the terms and conditions.
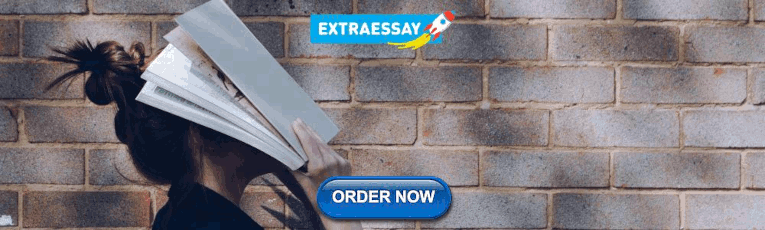
IMAGES
VIDEO
COMMENTS
Leeds LS2 9JT, UK. 3 Vice-Chancellor's O ce, University of Bath, Claverton Down, Bath BA2 7AY, UK. JE, 0000-0002-3319-9103. Optical wireless communication has attracted. significant interest ...
The major contributions of this thesis are: The design of a modular, low-cost, low-power, and high-speed underwater optical wireless transmitter using COTS electronic components. 1. The implementation of four common UOWC bitstream encodings on an FPGA. A comparison of the power consumption of each of the bitstream encodings.
Abstract: We reviewed the recent progress and applications of optical wireless communication (OWC), which includes optical camera communication (OCC) and visible light communication (VLC). We also summarized and discussed our recent works on OWC.
Abstract: The next generation wireless networks demand full coverage from the sky to the deep ocean, in which the water-air cross-boundary communication is a critical component in a coordinated uncrewed vehicular network that connects both uncrewed aerial vehicles (UAVs) and autonomous underwater vehicles (AUVs). In such a network, optical wireless communication (OWC) is an emerging and ...
The increasing demand for wireless networks of higher capacity requires key-enabling technologies. Optical wireless communication (OWC) arises as a complementary technology to radio frequency (RF) systems that can support high aggregate data rates. However, OWC systems face some challenges including beam-blockage. Intelligent reflecting surfaces (IRSs) can offer alternative pathways for the ...
Another important issue is the limited FOV of optical receivers. Thus, the impact of blockages in an RIS-assisted indoor LiFi communication system is investigated by taking into account the joint effect of optical receiver's limited FOV, user body blockage, and UE random orientation. iii. Declaration of originality.
Fig. 1: Attenuation of optical waves in aquatic medium. Due to the limitations of low bandwidth and low data rate of underwater acoustic and RF waves, an alternative approach is to use optical waves which can provide high-speed underwater optical wireless communication (UOWC) at low latencies in return for a limited communication range ...
Optical waves, on the other hand, have high bandwidth but they are a ected by other propagation e ects due to temperature changes, scattering, dispersion and beam wandering. Optical wireless underwater communication is limited to short distances due to severe water absorption at optical frequency band and strong back-scatter from suspended ...
In this thesis, optical communication using LEDs is presented as an improvement over acoustic modems for scenarios where high speed, but only moderate distances, is required and lower power, less complex communication systems are desired. ... Designing a wireless underwater optical communication system: en_US: dc.type: Thesis: en_US: dc ...
Underwater optical wireless communication (UOWC) is a technology that aims to apply high speed optical wireless communication (OWC) techniques to the underwater channel. ... This thesis first presents a method of modelling optical underwater turbulence through simulation that allows it to be examined in conjunction with absorption and ...
Optical wireless communication systems based on ultraviolet (UV)-band has a lot inherent advantages, such as low background solar radiation, low device dark noise. ... this thesis presents some efficient modulation schemes and available devices for the time being. Besides, a demonstration of ultraviolet-B (UVB) communication link is ...
High-speed and reliable underwater wireless optical communication system using multiple-input multiple-output and channel coding techniques for IoUT applications. Optics Communications , 461 : 1-8.
Optical Wireless Communication (OWC) is a line-of-sight wireless communication system in which data is transmitted in the form of laser beams. OWC is one of the best communication systems because of its high bandwidth, permissionless, highest transfer data rate, and ultra-high security. The development of OWC has increased extensively over the past decade, aided by the availability of powerful ...
Abstract: Optical wireless communication (OWC) is an excellent complementary solution to its radio frequency (RF) counterpart. OWC technologies have been demonstrated to be able to support high traffic generated by massive connectivity of the Internet of Things (IoT) and upcoming 5 th generation (5G) wireless communication systems. As the characteristics of OWC and RF are complementary, a ...
This thesis tries to improve the channel immunity by utilising optimised modulation to the channel by proposing an adaptive modulation technique, which can provide increased immunity against channel noise fluctuation at a relatively low complexity. High-speed wireless optical communication links have become more popular for personal mobile applications. This is a consequence of the increasing ...
Abstract. The emerging field of optical wireless communication (OWC) systems is seen as potential complementary technology to the radio frequency wireless communications in certain applications. It is deemed as a possible technology in the future 5th Generation communication networks to address the spectrum congestion and improve the system's ...
Recent advances in free-space optics have made outdoor optical wireless communication (OWC) an attractive solution to the "last-mile" problem of broadband access networks. Significant challenges can, however, arise for OWC links with increased levels of atmospheric turbulence from time-varying temperatures and pressures. As a promising alternative to the current generation of on-off keying ...
By combining this with satellite surface-chlorophyll data, it is possible to quantify the attenuation between any two locations in the ocean, with applications for low-noise or secure underwater communications and vertical links from the ocean surface. Depth variations in the attenuation coefficient for light in the ocean were calculated using a one-parameter model based on the chlorophyll-a ...
The purpose of this thesis is to lay the groundwork for the development of a cost-effective Underwater Optical Wireless Communications system. Currently, one of the largest barriers to the expansion of underwater enterprise and research is a lack of high-speed wireless communication systems. Wireless communication underwater
In 2015, Dr. Ismail had postdoctoral research in optical and wireless communications at the technical institute of microwave and photonic engineering, University of Graz, Austria. In 2018, he joined the optical wireless communication research group at the department of engineering and sciences, University of Oxford, UK to work in research of ...
Detailing a systems approach, Optical Wireless Communications: System and Channel Modelling with MATLAB, is a self-contained volume that concisely and comprehensively covers the theory and technology of optical wireless communications systems (OWC) in a way that is suitable for undergraduate and graduate-level students, as well as researchers and professional engineers.
Underwater wireless optical communication system under reciprocal turbulence Yujian Guo Underwater communication systems are in high demanded for subaquatic environment activities as the sea is an enormous and mostly unexplored place. The ten-meter long and few giga-bit per second range optical communication technique is feasible and has bright
In December 2014, the Task Group 802.15.7r1 (later named 802.15.m) 'Short Range Optical Wireless Communications' was formed. This task group was responsible for the development of both optical camera communication (OCC) and high-speed VLC where smartphone cameras and photodetectors are, respectively, used as receivers.
Underwater wireless communication refers to transmitting data in unguided water environment through the use of wireless carriers, i.e., radio-frequency wave, acoustic wave, and optical wave. We focus, in this thesis, on the underwater wireless optical communication (UWOC) that employs optical wave as the transmission carriers.
Optical wireless communication technologies such as free space optical communication, light fidelity, visible light communication, and optical camera communication can provide effective solutions for deployment of 6G systems and IoT [].Combination of free-space optical and mmWave systems provide significantly high data rates in wireless transmissions in spite of rapid variations in channel and ...
JAMSTEC has developed underwater optical wireless communication with a view to its use in underwater robots. Using a photomultiplier tube as a light receiving element and a wide light distribution LD as a light emitting element, a communication speed of 20 Mbps was achieved between underwater vehicles 120 m away. Furthermore, optical Wi-Fi is established between the autonomous underwater ...