Help | Advanced Search
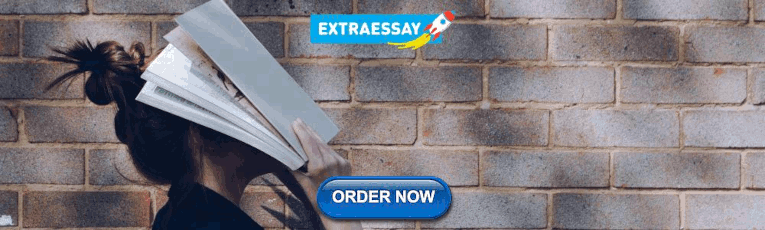
Physics > Applied Physics
Title: high-order harmonic generation from laser induced plasma comprising cdse/v2o5 core/shell quantum dots embedded on mos2 nanosheets.
Abstract: Research of the nonlinear optical characteristics of transition metal dichalcogenides in the presence of photoactive particles, plasmonic nanocavities, waveguides, and metamaterials is still in its early stages. This investigation delves into the high-order harmonic generation (HHG) from laser induced plasma of MoS2 nanosheets in the presence of semiconductor photoactive medium such as CdSe and CdSe/V2O5 core/shell quantum dots. Our comprehensive findings shed light on the counteractive coupling impact of both bare and passivated quantum dots on MoS2 nanosheets, as evidenced by the emission of higher-order harmonics. Significantly, the intensity of harmonics and their cut-off were notably enhanced in the MoS2-CdSe and MoS2-V-CdSe configurations compared to pristine MoS2 nanosheets. These advancements hold promise for applications requiring the emission of coherent short-wavelength radiation.
Submission history
Access paper:.
- Other Formats
References & Citations
- Google Scholar
- Semantic Scholar
BibTeX formatted citation

Bibliographic and Citation Tools
Code, data and media associated with this article, recommenders and search tools.
- Institution
arXivLabs: experimental projects with community collaborators
arXivLabs is a framework that allows collaborators to develop and share new arXiv features directly on our website.
Both individuals and organizations that work with arXivLabs have embraced and accepted our values of openness, community, excellence, and user data privacy. arXiv is committed to these values and only works with partners that adhere to them.
Have an idea for a project that will add value for arXiv's community? Learn more about arXivLabs .
Thank you for visiting nature.com. You are using a browser version with limited support for CSS. To obtain the best experience, we recommend you use a more up to date browser (or turn off compatibility mode in Internet Explorer). In the meantime, to ensure continued support, we are displaying the site without styles and JavaScript.
- View all journals
- Explore content
- About the journal
- Publish with us
- Sign up for alerts
- Published: 16 February 2024
Developing high-power Li||S batteries via transition metal/carbon nanocomposite electrocatalyst engineering
- Huan Li ORCID: orcid.org/0000-0003-0662-6939 1 na1 ,
- Rongwei Meng 2 na1 ,
- Chao Ye 1 ,
- Anton Tadich 3 ,
- Wuxing Hua ORCID: orcid.org/0000-0003-0385-3773 2 ,
- Qinfen Gu ORCID: orcid.org/0000-0001-9209-4208 3 ,
- Bernt Johannessen ORCID: orcid.org/0000-0002-3027-0816 3 , 4 ,
- Xiao Chen ORCID: orcid.org/0000-0003-1104-6146 5 ,
- Kenneth Davey 1 &
- Shi-Zhang Qiao ORCID: orcid.org/0000-0002-4568-8422 1
Nature Nanotechnology ( 2024 ) Cite this article
5469 Accesses
88 Altmetric
Metrics details
The activity of electrocatalysts for the sulfur reduction reaction (SRR) can be represented using volcano plots, which describe specific thermodynamic trends. However, a kinetic trend that describes the SRR at high current rates is not yet available, limiting our understanding of kinetics variations and hindering the development of high-power Li||S batteries. Here, using Le Chatelier’s principle as a guideline, we establish an SRR kinetic trend that correlates polysulfide concentrations with kinetic currents. Synchrotron X-ray adsorption spectroscopy measurements and molecular orbital computations reveal the role of orbital occupancy in transition metal-based catalysts in determining polysulfide concentrations and thus SRR kinetic predictions. Using the kinetic trend, we design a nanocomposite electrocatalyst that comprises a carbon material and CoZn clusters. When the electrocatalyst is used in a sulfur-based positive electrode (5 mg cm −2 of S loading), the corresponding Li||S coin cell (with an electrolyte:S mass ratio of 4.8) can be cycled for 1,000 cycles at 8 C (that is, 13.4 A g S −1 , based on the mass of sulfur) and 25 °C. This cell demonstrates a discharge capacity retention of about 75% (final discharge capacity of 500 mAh g S −1 ) corresponding to an initial specific power of 26,120 W kg S −1 and specific energy of 1,306 Wh kg S −1 .
This is a preview of subscription content, access via your institution
Access options
Access Nature and 54 other Nature Portfolio journals
Get Nature+, our best-value online-access subscription
24,99 € / 30 days
cancel any time
Subscribe to this journal
Receive 12 print issues and online access
251,40 € per year
only 20,95 € per issue
Buy this article
- Purchase on Springer Link
- Instant access to full article PDF
Prices may be subject to local taxes which are calculated during checkout
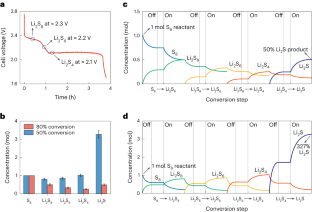
Similar content being viewed by others
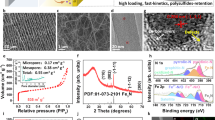
A Fe3N/carbon composite electrocatalyst for effective polysulfides regulation in room-temperature Na-S batteries
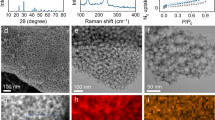
Enabling fast-charging selenium-based aqueous batteries via conversion reaction with copper ions
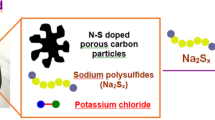
Straightforward synthesis of Sulfur/N,S-codoped carbon cathodes for Lithium-Sulfur batteries
Data availability.
Data supporting findings from this work are available within this Article and the Supplementary Information. All other relevant data supporting findings are available from the corresponding author on request. Source data are provided with this paper.
Peng, L. et al. A fundamental look at electrocatalytic sulfur reduction reaction. Nat. Catal. 3 , 762–770 (2020).
Article CAS Google Scholar
Zhao, C. et al. A high-energy and long-cycling lithium–sulfur pouch cell via a macroporous catalytic cathode with double-end binding sites. Nat. Nanotechnol. 16 , 166–173 (2020).
Article PubMed ADS Google Scholar
Zhao, C. X. et al. Semi-immobilized molecular electrocatalysts for high-performance lithium-sulfur batteries. J. Am. Chem. Soc. 143 , 19865–19872 (2021).
Article CAS PubMed Google Scholar
Zhou, G., Chen, H. & Cui, Y. Formulating energy density for designing practical lithium–sulfur batteries. Nat. Energy 7 , 312–319 (2022).
Article CAS ADS Google Scholar
Li, G., Chen, Z. & Lu, J. Lithium–sulfur batteries for commercial applications. Chem 4 , 3–7 (2018).
Manthiram, A., Fu, Y., Chung, S. H., Zu, C. & Su, Y. S. Rechargeable lithium–sulfur batteries. Chem. Rev. 114 , 11751–11787 (2014).
Fang, R. et al. More reliable lithium–sulfur batteries: status, solutions and prospects. Adv. Mater. 29 , 1606823 (2017).
Article Google Scholar
Pang, Q., Liang, X., Kwok, C. Y. & Nazar, L. F. Advances in lithium–sulfur batteries based on multifunctional cathodes and electrolytes. Nat. Energy 1 , 16132 (2016).
Tikekar, M. D., Choudhury, S., Tu, Z. Y. & Archer, L. A. Design principles for electrolytes and interfaces for stable lithium-metal batteries. Nat. Energy 1 , 16114–116120 (2016).
Yang, X., Luo, J. & Sun, X. Towards high-performance solid-state Li–S batteries: from fundamental understanding to engineering design. Chem. Soc. Rev. 49 , 2140–2195 (2020).
Yang, Y. et al. Electrocatalysis in lithium sulfur batteries under lean electrolyte conditions. Angew. Chem. Int. Ed. 57 , 15549–15552 (2018).
Song, Y. et al. Rationalizing electrocatalysis of Li–S chemistry by mediator design: progress and prospects. Adv. Energy Mater. 10 , 1901075 (2019).
Hua, W. et al. Selective catalysis remedies polysulfide shuttling in lithium–sulfur batteries. Adv. Mater. 33 , 2101006 (2021).
Dibden, J. W., Smith, J. W., Zhou, N., Garcia-Araez, N. & Owen, J. R. Predicting the composition and formation of solid products in lithium-sulfur batteries by using an experimental phase diagram. Chem. Commun. 52 , 12885–12888 (2016).
Shen, Z. et al. Cation-doped ZnS catalysts for polysulfide conversion in lithium–sulfur batteries. Nat. Catal. 5 , 555–563 (2022).
Zhong, Y. R. et al. Surface chemistry in cobalt phosphide-stabilized lithium–sulfur batteries. J. Am. Chem. Soc. 140 , 1455–1459 (2018).
Xue, W. et al. Intercalation-conversion hybrid cathodes enabling Li–S full-cell architectures with jointly superior gravimetric and volumetric energy densities. Nat. Energy 4 , 374–382 (2019).
Du, Z. et al. Cobalt in nitrogen-doped graphene as single-atom catalyst for high-sulfur content lithium–sulfur batteries. J. Am. Chem. Soc. 141 , 3977–3985 (2019).
Zhou, G. et al. Catalytic oxidation of Li 2 S on the surface of metal sulfides for Li–S batteries. Proc. Natl Acad. Sci. USA 114 , 840–845 (2017).
Article CAS PubMed PubMed Central ADS Google Scholar
De Heer, J. The principle of Le Châtelier and Braun. J. Chem. Educ. 34 , 375 (1957).
Zhang, L. et al. In situ optical spectroscopy characterization for optimal design of lithium–sulfur batteries. Chem. Soc. Rev. 48 , 5432–5453 (2019).
Li, H. et al. Revealing principles for design of lean-electrolyte lithium metal anode via in-situ spectroscopy. J. Am. Chem. Soc. 142 , 2012–2022 (2020).
Li, H. et al. Reversible electrochemical oxidation of sulfur in ionic liquid for high-voltage Al−S batteries. Nat. Commun. 12 , 5714 (2021).
Liu, L. & Corma, A. Metal catalysts for heterogeneous catalysis: from single atoms to nanoclusters and nanoparticles. Chem. Rev. 118 , 4981–5079 (2018).
Article CAS PubMed PubMed Central Google Scholar
Zou, Q. & Lu, Y. C. Solvent-dictated lithium sulfur redox reactions: an operando UV-vis spectroscopic study. J. Phys. Chem. Lett. 7 , 1518–1525 (2016).
Li, H. et al. Unraveling the catalyst-solvent interactions in lean-electrolyte sulfur reduction electrocatalysis for Li–S batteries. Angew. Chem. Int. Ed. 61 , e202213863 (2022).
He, Q., Freiberg, A. T. S., Patel, M. U. M., Qian, S. & Gasteiger, H. A. Operando identification of liquid intermediates in lithium–sulfur batteries via transmission UV–vis spectroscopy. J. Electrochem. Soc. 167 , 080508 (2020).
Hwang, J. et al. Perovskites in catalysis and electrocatalysis. Science 358 , 751–756 (2017).
Article CAS PubMed ADS Google Scholar
Singh, J. P., Park, J. Y., Chae, K. H., Ahn, D. & Lee, S. Soft X-ray absorption spectroscopic investigation of Li(Ni 0.8 Co 0.1 Mn 0.1 )O 2 cathode materials. Nanomaterials 10 , 759 (2020).
Massalski, T. B. & Okamoto, H. Binary Alloy Phase Diagram s 2nd edn (ASM International, 1990).
Kurata, H. & Colliex, C. Electron-energy-loss core-edge structures in manganese oxides. Phys. Rev. B 48 , 2102–2108 (1993).
Lin, F. et al. Synchrotron X-ray analytical techniques for studying materials electrochemistry in rechargeable batteries. Chem. Rev. 117 , 13123–13186 (2017).
Fan, F. Y., Carter, W. C. & Chiang, Y. M. Mechanism and kinetics of Li 2 S precipitation in lithium–sulfur batteries. Adv. Mater. 27 , 5203–5209 (2015).
Bhargav, A., He, J., Gupta, A. & Manthiram, A. Lithium–sulfur batteries: attaining the critical metrics. Joule 4 , 285–291 (2020).
Vivanco, J. P. & Rodriguez-Monroy, C. Graphene applications in the energy field: state-of-the-art and impact. In Proc. 16th LACCEI International Multi-Conference for Engineering, Education and Technology (2018).
Download references
Acknowledgements
This research was financially supported by the Australian Research Council (ARC) through the Discovery Project Program (grant numbers FL170100154 and DP220102596). Density functional theory computations were undertaken with the assistance of the National Computational Infrastructure (NCI), supported by the Australian Government. B.J. is supported by a Fellowship at the University of Wollongong. Part of this research was undertaken on the X-ray Absorption Spectroscopy (XAS), Powder Diffraction (PD) and Soft X-ray Spectroscopy (SXR) Beamlines at the Australian Synchrotron, part of ANSTO.
Author information
These authors contributed equally: Huan Li, Rongwei Meng.
Authors and Affiliations
School of Chemical Engineering, The University of Adelaide, Adelaide, South Australia, Australia
Huan Li, Chao Ye, Kenneth Davey & Shi-Zhang Qiao
School of Chemical Engineering and Technology, Tianjin University, Tianjin, China
Rongwei Meng & Wuxing Hua
Australian Synchrotron, ANSTO, Clayton, Victoria, Australia
Anton Tadich, Qinfen Gu & Bernt Johannessen
Institute for Superconducting and Electronic Materials, University of Wollongong, Wollongong, New South Wales, Australia
Bernt Johannessen
Beijing Key Laboratory of Green Reaction Engineering and Technology, Department of Chemical Engineering, Tsinghua University, Beijing, China
You can also search for this author in PubMed Google Scholar
Contributions
S.-Z.Q. conceived and supervised this research. H.L. designed and carried out experiments and density functional theory computations. R.M. carried out the synthesis of the cluster catalysts and electrochemical tests. C.Y. assisted with the design of the sulfur positive electrode. A.T. assisted with soft X-ray spectroscopy and related data analyses. W.H. tested the Li−S battery performance. Q.G. assisted with the in situ synchrotron X-ray diffraction measurements. B.J. carried out X-ray adsorption spectroscopy and data analyses. X.C. captured scanning transmission electron microscope images. S.-Z.Q. and K.D. revised the paper. All authors discussed the results and commented on the paper.
Corresponding author
Correspondence to Shi-Zhang Qiao .
Ethics declarations
Competing interests.
The authors declare no competing interests.
Peer review
Peer review information.
Nature Nanotechnology thanks Bing Joe Hwang and the other, anonymous, reviewer(s) for their contribution to the peer review of this work.
Additional information
Publisher’s note Springer Nature remains neutral with regard to jurisdictional claims in published maps and institutional affiliations.
Supplementary information
Supplementary information.
Supplementary Figs. 1–32, Tables 1 and 2, Notes 1–6 and References.
Source data
Source data fig. 1.
Source data for Fig. 1 are in sheet 1. Source Data Fig. 2 Source data for Fig. 2 are in sheet 2. Source Data Fig. 3 Source data for Fig. 3 are in sheet 3. Source Data Fig. 4 Source data for Fig. 4 are in sheet 4. Source Data Fig. 5 Source data for Fig. 5 are in sheet 5. Source Data Fig. 6 Source data for Fig. 6 are in sheet 6.
Rights and permissions
Springer Nature or its licensor (e.g. a society or other partner) holds exclusive rights to this article under a publishing agreement with the author(s) or other rightsholder(s); author self-archiving of the accepted manuscript version of this article is solely governed by the terms of such publishing agreement and applicable law.
Reprints and permissions
About this article
Cite this article.
Li, H., Meng, R., Ye, C. et al. Developing high-power Li||S batteries via transition metal/carbon nanocomposite electrocatalyst engineering. Nat. Nanotechnol. (2024). https://doi.org/10.1038/s41565-024-01614-4
Download citation
Received : 22 January 2023
Accepted : 19 January 2024
Published : 16 February 2024
DOI : https://doi.org/10.1038/s41565-024-01614-4
Share this article
Anyone you share the following link with will be able to read this content:
Sorry, a shareable link is not currently available for this article.
Provided by the Springer Nature SharedIt content-sharing initiative
Quick links
- Explore articles by subject
- Guide to authors
- Editorial policies
Sign up for the Nature Briefing newsletter — what matters in science, free to your inbox daily.

Physical Review Materials
- Research Updates
- Collections
- Editorial Team
Structural, magnetic, and magnetocaloric properties of triangular-lattice transition-metal phosphates
Chuandi zhang, junsen xiang, quanliang zhu, longfei wu, shanfeng zhang, juping xu, wen yin, peijie sun, wei li, gang su, and wentao jin, phys. rev. materials 8 , 044409 – published 26 april 2024.
- No Citing Articles
- INTRODUCTION
- RESULTS AND DISCUSSIONS
- ACKNOWLEDGMENTS
The recent discovery of the spin supersolid candidate Na 2 BaCo ( PO 4 ) 2 has stimulated a great deal of research on triangular-lattice transition-metal phosphates. Here we report a comprehensive study on the structural, magnetic, and magnetocaloric properties of polycrystalline Na 2 A T ( PO 4 ) 2 ( A = Ba,Sr; T = Co,Ni,Mn). X-ray and neutron diffraction measurements confirm that Na 2 Ba T ( PO 4 ) 2 ( NB T P ) crystallizes in a trigonal structure, while Na 2 Sr T ( PO 4 ) 2 ( NS T P ) forms a monoclinic structure with a slight distortion of the triangular network of T 2 + ions. The dc magnetization data show that all six compounds order antiferromagnetically below 2 K, and the Néel temperatures of NS T P are consistently higher than those of NB T P for T = Co, Ni, and Mn, due to the release of geometrical frustration by monoclinical distortions. Furthermore, magnetocaloric measurements show that trigonal NB T P can reach a lower temperature in the quasiadiabatic demagnetization process and thus it demonstrates a better performance in the magnetic refrigeration, compared with monoclinic NS T P . Our findings highlight the outstanding magnetocaloric performances of the trigonal transition-metal phosphates and disclose two necessary ingredients for a superior magnetic coolant that can reach an ultralow temperature, including a perfect geometrically frustrated lattice and a small effective spin number associated with the magnetic ions.
- Received 17 January 2024
- Accepted 1 April 2024
DOI: https://doi.org/10.1103/PhysRevMaterials.8.044409
©2024 American Physical Society
Physics Subject Headings (PhySH)
- Research Areas
- Physical Systems
Authors & Affiliations
- 1 School of Physics, Beihang University, Beijing 100191, China
- 2 Beijing National Laboratory for Condensed Matter Physics, Institute of Physics, Chinese Academy of Sciences, 100190 Beijing, China
- 3 Spallation Neutron Source Science Center, Dongguan 523803, China
- 4 CAS Key Laboratory of Theoretical Physics, Institute of Theoretical Physics, Chinese Academy of Sciences, Beijing 100190, China
- 5 CAS Center for Excellence in Topological Quantum Computation, University of Chinese Academy of Sciences, Beijing 100190, China
- 6 Peng Huanwu Collaborative Center for Research and Education, Beihang University, Beijing 100191, China
- 7 Kavli Institute for Theoretical Sciences, and School of Physical Sciences, University of Chinese Academy of Sciences, Beijing 100049, China
- * These authors contributed equally to this work.
- † [email protected]
Article Text (Subscription Required)
References (subscription required).
Vol. 8, Iss. 4 — April 2024
Access Options
- Buy Article »
- Log in with individual APS Journal Account »
- Log in with a username/password provided by your institution »
- Get access through a U.S. public or high school library »
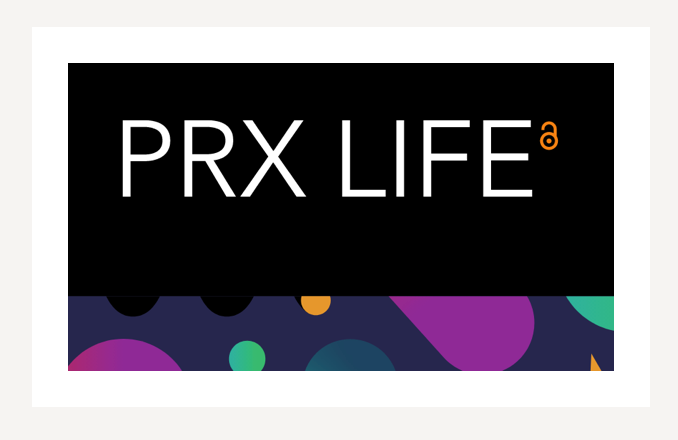
Authorization Required
Other options.
- Buy Article »
- Find an Institution with the Article »
Download & Share
Room-temperature XRD and NPD patterns of NBNP (a), (e), NBMP (b), (f), NSNP (c), (g), and NSMP (d), (h) and corresponding Rietveld refinements. The circles represent the observed intensities, and the solid lines are the calculated patterns. The differences between the observed and calculated intensities are shown at the bottom. The short vertical bars correspond to the expected nuclear Bragg reflections.
The monoclinic unit cell of NS T P (a) with the in-plane structure viewed along the c axis illustrated in (b), where the angle enclosed by the dashed line marks the in-plane T − T − T bond angle in the isosceles triangular network of the T 2 + layers.
dc magnetic susceptibility ( χ , filled circles) and inverse susceptibility (1/ χ , empty squares) of the polycrystalline NBCP (a), NBNP (b), NBMP (c), NSCP (d), NSNP (e), and NSMP (f), respectively, measured in a magnetic field of 0.1 T. The black solid lines represent the CW fittings to 1/ χ in the low-temperature paramagnetic state from 2 to 20 K. The insets are enlarged plots of χ ( T ) in the temperature range from 0.4 to 1.8 K, in which the arrows mark the AFM ordering temperatures.
Isothermal magnetization [ M ( H ) , filled circles] of polycrystalline NBCP (a), NBNP (b), NBMP (c), NSCP (d), NSNP (e), and NSMP (f), respectively, measured at 0.4 K and their first derivatives ( d M / d H , solid lines). Dashed lines in (a), (b), and (d) correspond to the contribution from the Van-Vleck paramagnetism. The shaded zones in (b) and (c) mark the region of the slope change of the M ( H ) curve, for which a dip appears in d M / d H for NBNP and NBMP. The green arrows in (a), (b), and (d) mark the critical field ( H s ) corresponding to the moment saturation for NBCP, NBNP, and NSCP, respectively.
The cooling curve of polycrystalline Na 2 A T ( PO 4 ) 2 samples in the adiabatic demagnetization process starting from an initial temperature of T 0 = 2 K and an initial magnetic field of H 0 = 4 T (a) and 6 T (b), respectively. The cooling performances of the commercial sub-Kelvin magnetic coolant GGG in similar conditions are also shown, in dashed lines, for comparison.
The zero-field molar specific heat ( C , filled circles) and magnetic entropy ( S , solid lines) of NBCP and GGG single crystals, respectively, at low temperatures. The specific-heat data of single-crystal GGG are from Refs. [ 46, 47 ]. S is obtained by integrating C / T . The horizontal dashed lines mark the maximal magnetic entropy of R ln2 and R ln8 as expected for the J eff = 1/2 and J = 7/2 systems, respectively.
Sign up to receive regular email alerts from Physical Review Materials
- Forgot your username/password?
- Create an account
Article Lookup
Paste a citation or doi, enter a citation.
Advertisement
Recent developments in transition metal-based nanomaterials for supercapacitor applications
- FOCUS ISSUE: Transition Metal-based Nanomaterials
- Published: 02 June 2022
- Volume 37 , pages 2124–2149, ( 2022 )
Cite this article
- Rahul Singhal ORCID: orcid.org/0000-0002-5017-9091 1 ,
- Manika Chaudhary 2 ,
- Shrestha Tyagi 2 ,
- Deepanshi Tyagi 2 ,
- Vanshika Bhardwaj 2 &
- Beer Pal Singh 2
1037 Accesses
11 Citations
Explore all metrics
In the recent years the demand of high energy density, high power density energy storage device with long cycle stability increased because of their vast applications from portable electronics devices to power tolls and hybrid electric vehicles. Also, the developments in renewable energy sources also created immediate demand for high energy density energy storage devices. Supercapacitors are found to be suitable to fulfill the current demand of energy storage devices. Transition metal nanomaterials are considered to store high charge because of their large surface area and variable oxidation states. In the present review, we discussed the recent advances in the area of supercapacitors using transition metal oxides, nitride, sulfides, diselenides, phosphides, and ferrites. The effect of surface morphology, synthesis process, and various doping/composites on specific capacitance of supercapacitors were discussed in detail.
Graphical abstract
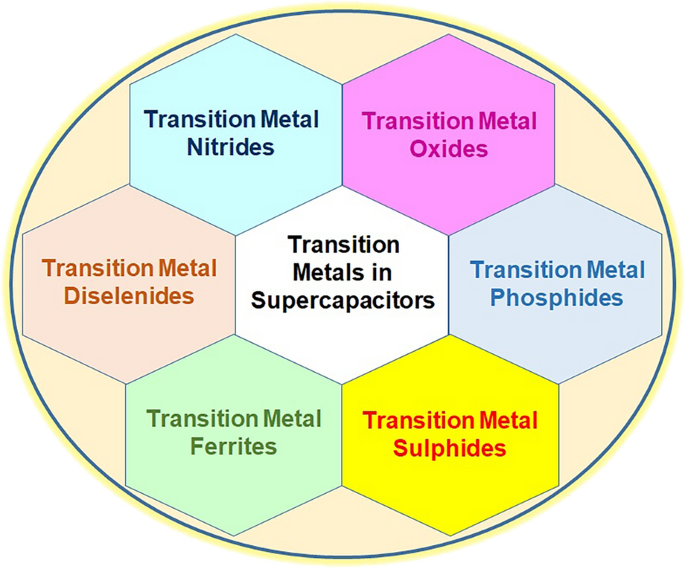
This is a preview of subscription content, log in via an institution to check access.
Access this article
Price includes VAT (Russian Federation)
Instant access to the full article PDF.
Rent this article via DeepDyve
Institutional subscriptions
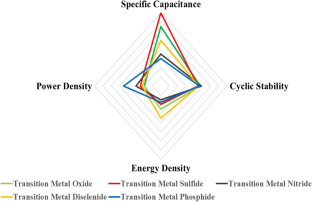
Similar content being viewed by others
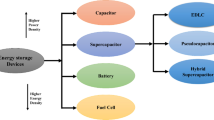
A review on electrolytes for supercapacitor device
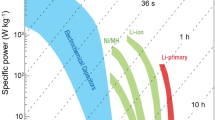
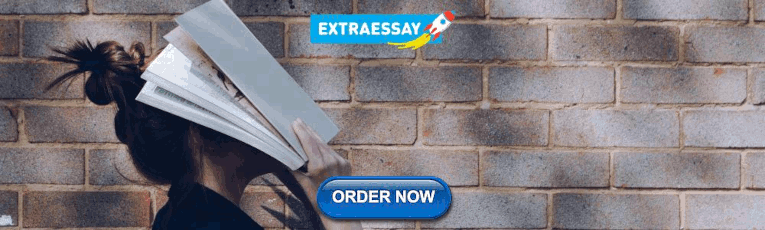
Recent progress in carbon-based materials for supercapacitor electrodes: a review
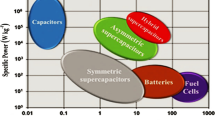
Advances in Supercapacitor Development: Materials, Processes, and Applications
G. Wang, L. Zhang, J. Zhang, A review of electrode materials for electrochemical supercapacitors. Chem. Soc. Rev. 41 (2), 797–828 (2012). https://doi.org/10.1039/C1CS15060J
Article CAS Google Scholar
X. Wang, G. Pawar, Y. Li, X. Ren, M. Zhang, B. Lu, A. Banerjee, P. Liu, E.J. Dufek, J.-G. Zhang, Glassy Li metal anode for high-performance rechargeable Li batteries. Nat. Mater. 19 (12), 1339–1345 (2020). https://doi.org/10.1038/s41563-020-0729-1
Y. Liu, X. Li, W. Shen, Y. Dai, W. Kou, W. Zheng, X. Jiang, G. He, Multishelled transition metal-based microspheres: synthesis and applications for batteries and supercapacitors. Small 15 (32), 1804737 (2019). https://doi.org/10.1002/smll.201804737
M.D. Hager, B. Esser, X. Feng, W. Schuhmann, P. Theato, U.S. Schubert, Polymer-based batteries—flexible and thin energy storage systems. Adv. Mater. 32 (39), 2000587 (2020). https://doi.org/10.1002/adma.202000587
H. Jin, S. Dai, K. Xie, Y. Luo, K. Liu, Z. Zhu, L. Huang, L. Huang, J. Zhou, Regulating interfacial desolvation and deposition kinetics enables durable Zn anodes with ultrahigh utilization of 80%. Small 18 (4), 2106441 (2022). https://doi.org/10.1002/smll.202106441
Y. Zhou, C.H. Wang, W. Lu, L. Dai, Fiber-shaped energy-storage devices: recent advances in fiber-shaped supercapacitors and lithium-ion batteries. Adv. Mater. 32 (5), 2070037 (2020). https://doi.org/10.1002/adma.202070037
Article Google Scholar
S. Zheng, X. Shi, P. Das, Z.S. Wu, X. Bao, Microscale energy-storage devices: the road towards planar microbatteries and micro-supercapacitors: from 2D to 3D device geometries. Adv. Mater. 31 (50), 1970351 (2019). https://doi.org/10.1002/adma.201970351
F. Béguin, V. Presser, A. Balducci, E. Frackowiak, Carbons and electrolytes for advanced supercapacitors. Adv. Mater. 26 (14), 2219–2251 (2014). https://doi.org/10.1002/adma.201304137
F. Wu, H. Yang, Y. Bai, C. Wu, Paving the path toward reliable cathode materials for aluminum-ion batteries. Adv. Mater. 31 (16), 1806510 (2019). https://doi.org/10.1002/adma.201806510
A. Konarov, N. Voronina, J.H. Jo, Z. Bakenov, Y.-K. Sun, S.-T. Myung, Present and future perspective on electrode materials for rechargeable zinc-ion batteries. ACS Energy Lett. 3 (10), 2620–2640 (2018). https://doi.org/10.1021/acsenergylett.8b01552
S. Yang, S. Wang, X. Liu, L. Li, Biomass derived interconnected hierarchical micro-meso-macro-porous carbon with ultrahigh capacitance for supercapacitors. Carbon 147 , 540–549 (2019). https://doi.org/10.1016/j.carbon.2019.03.023
Y. Zhang, S. Yang, S. Wang, X. Liu, L. Li, Microwave/freeze casting assisted fabrication of carbon frameworks derived from embedded upholder in tremella for superior performance supercapacitors. Energy Storage Materials 18 , 447–455 (2019). https://doi.org/10.1016/j.ensm.2018.08.006
R. Dubey, V. Guruviah, Review of carbon-based electrode materials for supercapacitor energy storage. Ionics 25 (4), 1419–1445 (2019). https://doi.org/10.1007/s11581-019-02874-0
L. Wang, L. Wen, Y. Tong, S. Wang, X. Hou, X. An, S.X. Dou, J. Liang, Photo-rechargeable batteries and supercapacitors: Critical roles of carbon-based functional materials. Carbon Energy 3 (2), 225–252 (2021). https://doi.org/10.1002/cey2.105
A. Riaz, M.R. Sarker, M.H.M. Saad, R. Mohamed, Review on comparison of different energy storage technologies used in micro-energy harvesting, WSNs, low-cost microelectronic devices: challenges and recommendations. Sensors (Basel) (2021). https://doi.org/10.3390/s21155041
A. Borenstein, O. Hanna, R. Attias, S. Luski, T. Brousse, D. Aurbach, Carbon-based composite materials for supercapacitor electrodes: a review. J. Mater. Chem. A 5 (25), 12653–12672 (2017). https://doi.org/10.1039/C7TA00863E
A. González, E. Goikolea, J.A. Barrena, R. Mysyk, Review on supercapacitors: technologies and materials. Renew. Sustain. Energy Rev. 58 , 1189–1206 (2016). https://doi.org/10.1016/j.rser.2015.12.249
A. Berrueta, A. Ursúa, I. San Martín, A. Eftekhari, P. Sanchis, Supercapacitors: electrical characteristics, modeling, applications, and future trends. IEEE Access 7 , 50869–50896 (2019). https://doi.org/10.1109/ACCESS.2019.2908558
J. Wang, J. Wang, Z. Kong, K. Lv, C. Teng, Y. Zhu, Conducting-polymer-based materials for electrochemical energy conversion and storage. Adv. Mater. 29 (45), 1703044 (2017). https://doi.org/10.1002/adma.201703044
W. Raza, F. Ali, N. Raza, Y. Luo, K.-H. Kim, J. Yang, S. Kumar, A. Mehmood, E.E. Kwon, Recent advancements in supercapacitor technology. Nano Energy 52 , 441–473 (2018). https://doi.org/10.1016/j.nanoen.2018.08.013
Z. Qiu, Y. Wang, X. Bi, T. Zhou, J. Zhou, J. Zhao, Z. Miao, W. Yi, P. Fu, S. Zhuo, Biochar-based carbons with hierarchical micro-meso-macro porosity for high rate and long cycle life supercapacitors. J. Power Sources 376 , 82–90 (2018). https://doi.org/10.1016/j.jpowsour.2017.11.077
K. Ren, Z. Liu, T. Wei, Z. Fan, Recent developments of transition metal compounds-carbon hybrid electrodes for high energy/power supercapacitors. Nano-Micro Lett. 13 (1), 1–32 (2021). https://doi.org/10.1007/s40820-021-00642-2
P. Veerakumar, A. Sangili, S. Manavalan, P. Thanasekaran, K.-C. Lin, Research progress on porous carbon supported metal/metal oxide nanomaterials for supercapacitor electrode applications. Ind. Eng. Chem. Res. 59 (14), 6347–6374 (2020). https://doi.org/10.1021/acs.iecr.9b06010
K.A. Owusu, L. Qu, J. Li, Z. Wang, K. Zhao, C. Yang, K.M. Hercule, C. Lin, C. Shi, Q. Wei, Low-crystalline iron oxide hydroxide nanoparticle anode for high-performance supercapacitors. Nat. Commun. 8 (1), 1–11 (2017). https://doi.org/10.1038/ncomms14264
L. Zheng, J. Song, X. Ye, Y. Wang, X. Shi, H. Zheng, Construction of self-supported hierarchical NiCo-S nanosheet arrays for supercapacitors with ultrahigh specific capacitance. Nanoscale 12 (25), 13811–13821 (2020). https://doi.org/10.1039/D0NR02976A
P. Geng, S. Zheng, H. Tang, R. Zhu, L. Zhang, S. Cao, H. Xue, H. Pang, Transition metal sulfides based on graphene for electrochemical energy storage. Adv. Energy Mater. 8 (15), 1703259 (2018)
Y. Wang, Y. Song, Y. Xia, Electrochemical capacitors: mechanism, materials, systems, characterization and applications. Chem. Soc. Rev. 45 (21), 5925–5950 (2016). https://doi.org/10.1039/C5CS00580A
H. Liu, X. Liu, S. Wang, H.-K. Liu, L. Li, Transition metal based battery-type electrodes in hybrid supercapacitors: a review. Energy Storage Materials 28 , 122–145 (2020). https://doi.org/10.1016/j.ensm.2020.03.003
L. Hou, W. Yang, R. Li, X. Xu, P. Wang, B. Deng, F. Yang, Y. Li, Self-reconstruction strategy to synthesis of Ni/Co-OOH nanoflowers decorated with N, S co-doped carbon for high-performance energy storage. Chem. Eng. J. 396 , 125323 (2020). https://doi.org/10.1016/j.cej.2020.125323
Y. Son, M. Park, Y. Son, J.-S. Lee, J.-H. Jang, Y. Kim, J. Cho, Quantum confinement and its related effects on the critical size of GeO2 nanoparticles anodes for lithium batteries. Nano Lett. 14 (2), 1005–1010 (2014). https://doi.org/10.1021/nl404466v
R. Mo, Z. Lei, K. Sun, D. Rooney, Facile synthesis of anatase TiO2 quantum-dot/graphene-nanosheet composites with enhanced electrochemical performance for lithium-ion batteries. Adv. Mater. 26 (13), 2084–2088 (2014). https://doi.org/10.1002/adma.201304338
H. Xia, C. Hong, B. Li, B. Zhao, Z. Lin, M. Zheng, S.V. Savilov, S.M. Aldoshin, Facile synthesis of hematite quantum-dot/functionalized graphene-sheet composites as advanced anode materials for asymmetric supercapacitors. Adv. Func. Mater. 25 (4), 627–635 (2015). https://doi.org/10.1002/adfm.201403554
H.M. Jeong, K.M. Choi, T. Cheng, D.K. Lee, R. Zhou, I.W. Ock, D.J. Milliron, W.A. Goddard, J.K. Kang, Rescaling of metal oxide nanocrystals for energy storage having high capacitance and energy density with robust cycle life. Proc. Natl. Acad. Sci. U.S.A. 112 (26), 7914–7919 (2015). https://doi.org/10.1073/pnas.1503546112
N. Agnihotri, P. Sen, A. De, M. Mukherjee, Hierarchically designed PEDOT encapsulated graphene-MnO2 nanocomposite as supercapacitors. Mater. Res. Bull. 88 , 218–225 (2017). https://doi.org/10.1016/j.materresbull.2016.12.036
G. Wang, Z. Jin, Q. Guo, Ordered self-supporting NiV LDHs@ P-nickel foam nano-array as high-performance supercapacitor electrode. J. Colloid Interface Sci. 583 , 1–12 (2021). https://doi.org/10.1016/j.jcis.2020.08.127
G. Zhang, T. Wu, H. Zhou, H. Jin, K. Liu, Y. Luo, H. Jiang, K. Huang, L. Huang, J. Zhou, Rich alkali ions preintercalated vanadium oxides for durable and fast zinc-ion storage. ACS Energy Lett. 6 (6), 2111–2120 (2021). https://doi.org/10.1021/acsenergylett.1c00625
W. Wu, L. Yang, S. Chen, Y. Shao, L. Jing, G. Zhao, H. Wei, Core–shell nanospherical polypyrrole/graphene oxide composites for high performance supercapacitors. RSC Adv. 5 (111), 91645–91653 (2015). https://doi.org/10.1039/C5RA17036B
Z. Wang, Y. Long, D. Cao, D. Han, F. Gu, A high-performance flexible supercapacitor based on hierarchical Co3O4-SnO@SnO2 nanostructures. Electrochim. Acta 307 , 341–350 (2019). https://doi.org/10.1016/j.electacta.2019.03.230
M. Chaudhary, M. Singh, A. Kumar, Prachi, Y.K. Gautam, A.K. Malik, Y. Kumar, and B.P. Singh, Experimental investigation of Co and Fe-Doped CuO nanostructured electrode material for remarkable electrochemical performance. Ceram. Int. 47 (2), 2094–2106. (2021). https://doi.org/10.1016/j.ceramint.2020.09.042 .
J. Wan, X. Yao, X. Gao, X. Xiao, T. Li, J. Wu, W. Sun, Z. Hu, H. Yu, L. Huang, M. Liu, J. Zhou, Microwave combustion for modification of transition metal oxides. Adv. Func. Mater. 26 (40), 7263–7270 (2016). https://doi.org/10.1002/adfm.201603125
A.C. Nwanya, D. Obi, K.I. Ozoemena, R.U. Osuji, C. Awada, A. Ruediger, M. Maaza, F. Rosei, F.I. Ezema, Facile synthesis of nanosheet-like CuO film and its potential application as a high-performance pseudocapacitor electrode. Electrochim. Acta 198 , 220–230 (2016). https://doi.org/10.1016/j.electacta.2016.03.064
S. Paulraj, R. Jayavel, Microwave-assisted synthesis of Ru and Ce doped tungsten oxide for supercapacitor electrodes. J. Mater. Sci.: Mater. Electron. 29 (16), 13794–13802 (2018). https://doi.org/10.1007/s10854-018-9510-5
Y. Wang, X. Li, Y. Wang, Y. Liu, Y. Bai, R. Liu, G. Yuan, High-performance flexible MnO2@carbonized cotton textile electrodes for enlarged operating potential window symmetrical supercapacitors. Electrochim. Acta 299 , 12–18 (2019). https://doi.org/10.1016/j.electacta.2018.12.181
L. Cui, C. Cheng, F. Peng, Y. Yang, Y. Li, M. Jia, X. Jin, A ternary MnO2-deposited RGO/lignin-based porous carbon composite electrode for flexible supercapacitor applications. New J. Chem. 43 (35), 14084–14092 (2019). https://doi.org/10.1039/c9nj02184a
M. Ghorbani, M.R. Golobostanfard, H. Abdizadeh, Flexible freestanding sandwich type ZnO/rGO/ZnO electrode for wearable supercapacitor. Appl. Surf. Sci. 419 , 277–285 (2017). https://doi.org/10.1016/j.apsusc.2017.05.060
Y. Guo, Z. Zhu, Y. Chen, H. He, X. Li, T. Qin, Y. Wang, High-performance supercapacitors of ruthenium-based nanohybrid compounds. J. Alloy Compd. 842 , 155798 (2020). https://doi.org/10.1016/j.jallcom.2020.155798
K.-C. Huang, C.-H. Lin, K.S. Anuratha, T.-Y. Huang, J.-Y. Lin, F.-G. Tseng, C.-K. Hsieh, Laser printer patterned sacrificed layer for arbitrary design and scalable fabrication of the all-solid-state interdigitated in-planar hydrous ruthenium oxide flexible micro supercapacitors. J. Power Sources 417 , 108–116 (2019). https://doi.org/10.1016/j.jpowsour.2019.02.016
S.N. Khatavkar, S.D. Sartale, α-Fe2O3 thin film on stainless steel mesh: a flexible electrode for supercapacitor. Mater. Chem. Phys. 225 , 284–291 (2019). https://doi.org/10.1016/j.matchemphys.2018.12.079
J. Shen, Q. Wang, K. Zhang, S. Wang, L. Li, S. Dong, S. Zhao, J. Chen, R. Sun, Y. Wang, Z. Jian, W. Zhang, Flexible carbon cloth based solid-state supercapacitor from hierarchical holothurian-morphological NiCo2O4@NiMoO4/PANI. Electrochim. Acta 320 , 134578 (2019). https://doi.org/10.1016/j.electacta.2019.134578
S. Liu, Y. Yin, Y. Shen, K.S. Hui, Y.T. Chun, J.M. Kim, K.N. Hui, L. Zhang, S.C. Jun, Phosphorus regulated cobalt oxide@nitrogen-doped carbon nanowires for flexible quasi-solid-state supercapacitors. Small 16 (4), 1906458 (2020). https://doi.org/10.1002/smll.201906458
X. Li, Z. Yang, W. Qi, Y. Li, Y. Wu, S. Zhou, S. Huang, J. Wei, H. Li, P. Yao, Binder-free Co3O4@NiCoAl-layered double hydroxide core-shell hybrid architectural nanowire arrays with enhanced electrochemical performance. Appl. Surf. Sci. 363 , 381–388 (2016). https://doi.org/10.1016/j.apsusc.2015.12.039
S.C. Lee, M. Kim, J.-H. Park, E.S. Kim, S. Liu, K.Y. Chung, S. Chan Jun, An unexpected phase-transformation of cobalt–vanadium layered double hydroxides toward high energy density hybrid supercapacitor. J Power Sources 486 , 229–341 (2021). https://doi.org/10.1016/j.jpowsour.2020.229341
P.A. Shinde, N.R. Chodankar, S. Lee, E. Jung, S. Aftab, Y.-K. Han, S.C. Jun, All-redox solid-state supercapacitor with cobalt manganese oxide@bimetallic hydroxides and vanadium nitride@nitrogen-doped carbon electrodes. Chem. Eng. J. 405 , 127029 (2021). https://doi.org/10.1016/j.cej.2020.127029
X. Tang, X. Guo, W. Wu, G. Wang, 2D metal carbides and nitrides (MXenes) as high-performance electrode materials for Lithium-based batteries. Adv. Energy Mater. 8 (33), 1801897 (2018). https://doi.org/10.1002/aenm.201801897
C. Zhu, Y. Sun, D. Chao, X. Wang, P. Yang, X. Zhang, H. Huang, H. Zhang, H.J. Fan, A 2.0 V capacitive device derived from shape-preserved metal nitride nanorods. Nano Energy 26 , 1–6 (2016). https://doi.org/10.1016/j.nanoen.2016.04.056
Y. Zhang, B. Ouyang, J. Xu, G. Jia, S. Chen, R.S. Rawat, H.J. Fan, Rapid synthesis of cobalt nitride nanowires: highly efficient and low-cost catalysts for oxygen evolution. Angew. Chem. 128 (30), 8812–8816 (2016). https://doi.org/10.1002/ange.201604372
B. Gao, X. Li, K. Ding, C. Huang, Q. Li, P.K. Chu, K. Huo, Recent progress in nanostructured transition metal nitrides for advanced electrochemical energy storage. J. Mater. Chem. A 7 (1), 14–37 (2019). https://doi.org/10.1039/C8TA05760E
Y. Zhong, X. Xia, F. Shi, J. Zhan, J. Tu, H.J. Fan, Transition metal carbides and nitrides in energy storage and conversion. Adv. Sci. 3 (5), 150286 (2016)
M.-S. Balogun, Y. Huang, W. Qiu, H. Yang, H. Ji, Y. Tong, Updates on the development of nanostructured transition metal nitrides for electrochemical energy storage and water splitting. Mater. Today 20 (8), 425–451 (2017)
D. Choi, P.N. Kumta, Synthesis and characterization of nanostructured niobium and molybdenum nitrides by a two-step transition metal halide approach. J. Am. Ceram. Soc. 94 (8), 2371–2378 (2011). https://doi.org/10.1111/j.1551-2916.2011.04412.x
K. Schwarz, Band structure and chemical bonding in transition metal carbides and nitrides. Crit. Rev. Solid State Mater. Sci. 13 (3), 211–257 (1987). https://doi.org/10.1080/10408438708242178
D.J. Ham, J.S. Lee, Transition metal carbides and nitrides as electrode materials for low temperature fuel cells. Energies 2 (4), 873–899 (2009). https://doi.org/10.3390/en20400873
D. Zhao, Z. Cui, S. Wang, J. Qin, M. Cao, VN hollow spheres assembled from porous nanosheets for high-performance lithium storage and the oxygen reduction reaction. J. Mater. Chem. A 4 (20), 7914–7923 (2016). https://doi.org/10.1039/C6TA01707J
C. Huang, Y. Yang, J. Fu, J. Wu, H. Song, X. Zhang, B. Gao, P.K. Chu, K. Huo, Flexible Nb4N5/rGO electrode for high-performance solid state supercapacitors. J. Nanosci. Nanotechnol. 18 (1), 30–38 (2018). https://doi.org/10.1166/jnn.2018.14595
W. Bi, Z. Hu, X. Li, C. Wu, J. Wu, Y. Wu, Y. Xie, Metallic mesocrystal nanosheets of vanadium nitride for high-performance all-solid-state pseudocapacitors. Nano Res. 8 (1), 193–200 (2015). https://doi.org/10.1007/s12274-014-0612-y
C. Dong, X. Wang, X. Liu, X. Yuan, W. Dong, H. Cui, Y. Duan, F. Huang, In situ grown Nb4N5 nanocrystal on nitrogen-doped graphene as a novel anode for lithium ion battery. RSC Adv. 6 (84), 81290–81295 (2016). https://doi.org/10.1039/c6ra13647h
H. Xu, H. Zhang, L. Fang, J. Yang, K. Wu, Y. Wang, Hierarchical molybdenum nitride nanochexes by a textured self-assembly in gas–solid phase for the enhanced application in lithium ion batteries. ACS Nano 9 (7), 6817–6825 (2015). https://doi.org/10.1021/acsnano.5b02415
B. Das, M. Behm, G. Lindbergh, M.V. Reddy, B.V.R. Chowdari, High performance metal nitrides, MN (M = Cr, Co) nanoparticles for non-aqueous hybrid supercapacitors. Adv. Powder Technol. 26 (3), 783–788 (2015). https://doi.org/10.1016/j.apt.2015.02.001
X. Jiang, W. Lu, X. Yu, S. Song, Y. Xing, Fabrication of a vanadium nitride/N-doped carbon hollow nanosphere composite as an efficient electrode material for asymmetric supercapacitors. Nanoscale Adv. 2 (9), 3865–3871 (2020). https://doi.org/10.1039/D0NA00288G
Z. Qi, B. Wei, J. Wang, Y. Yang, Z. Wang, Nanostructured porous CrN thin films by oblique angle magnetron sputtering for symmetric supercapacitors. J. Alloy Compd. 806 , 953–959 (2019). https://doi.org/10.1016/j.jallcom.2019.07.325
A. Guerra, E. Haye, A. Achour, M. Harnois, T. Hadjersi, J.-F. Colomer, J.-J. Pireaux, S. Lucas, R. Boukherroub, High performance of 3D silicon nanowires array@CrN for electrochemical capacitors. Nanotechnology 31 (3), 035407 (2020). https://doi.org/10.1088/1361-6528/ab4963
Z. Gao, Z. Wu, S. Zhao, T. Zhang, Q. Wang, Enhanced capacitive property of HfN film electrode by plasma etching for supercapacitors. Mater. Lett. 235 , 148–152 (2019). https://doi.org/10.1016/j.matlet.2018.10.032
H. Shen, B. Wei, D. Zhang, Z. Qi, Z. Wang, Magnetron sputtered NbN thin film electrodes for supercapacitors. Mater. Lett. 229 , 17–20 (2018). https://doi.org/10.1016/j.matlet.2018.06.052
T. He, W. Zhang, P. Manasa, F. Ran, Quantum dots of molybdenum nitride embedded in continuously distributed polyaniline as novel electrode material for supercapacitor. J. Alloy Compd. 812 , 152138 (2020). https://doi.org/10.1016/j.jallcom.2019.152138
S. Ouendi, K. Robert, D. Stiévenard, T. Brousse, P. Roussel, C. Lethien, Sputtered tungsten nitride films as pseudocapacitive electrode for on chip micro-supercapacitors. Energy Storage Mater. 20 , 243–252 (2019). https://doi.org/10.1016/j.ensm.2019.04.006
X. Xu, S. Chang, Z. Hong, Y. Zeng, H. Zhang, P. Li, S. Zheng, Z. Wang, S. Duo, Construction of 3D CrN@ nitrogen-doped carbon nanosheet arrays by reactive magnetron sputtering for the free-standing electrode of supercapacitor. Nanotechnology 33 (5), 055402 (2021). https://doi.org/10.1088/1361-6528/ac3356
S. Venkateshalu, J. Cherusseri, M. Karnan, K.S. Kumar, P. Kollu, M. Sathish, J. Thomas, S.K. Jeong, A.N. Grace, New method for the synthesis of 2D vanadium nitride (MXene) and its application as a supercapacitor electrode. ACS Omega 5 (29), 17983–17992 (2020). https://doi.org/10.1021/acsomega.0c01215
L. Xu, L. Sun, J. Feng, L. Qi, I. Muhammad, J. Maher, X. Cheng, W. Song, Nanocasting synthesis of an iron nitride-ordered mesopore carbon composite as a novel electrode material for supercapacitors. RSC Adv. 7 (70), 44619–44625 (2017). https://doi.org/10.1039/c7ra08704g
A. Śliwak, A. Moyseowicz, G. Gryglewicz, Hydrothermal-assisted synthesis of an iron nitride–carbon composite as a novel electrode material for supercapacitors. J. Mater. Chem. A 5 (12), 5680–5684 (2017). https://doi.org/10.1039/C6TA10985C
M. Ishaq, M. Jabeen, W. Song, L. Xu, W. Li, Q. Deng, Fluorinated graphene-supported Nickel-Cobalt-Iron nitride nanoparticles as a promising hybrid electrode for supercapacitor applications. Electrochim. Acta 282 , 913–922 (2018). https://doi.org/10.1016/j.electacta.2018.06.087
N. Ouldhamadouche, A. Achour, R. Lucio-Porto, M. Islam, S. Solaymani, A. Arman, A. Ahmadpourian, H. Achour, L. Le Brizoual, M.A. Djouadi, Electrodes based on nano-tree-like vanadium nitride and carbon nanotubes for micro-supercapacitors. J. Mater. Sci. Technol. 34 (6), 976–982 (2018). https://doi.org/10.1016/j.jmst.2017.11.048
W. Zhang, Y. Yang, M. Ravi, L. Kong, L. Kang, F. Ran, Interconnected porous composites electrode materials of Carbon@Vanadium nitride by directly absorbing VO 3 . Electrochim. Acta 306 , 113–121 (2019). https://doi.org/10.1016/j.electacta.2019.03.112
A. Salman, S. Padmajan Sasikala, I.H. Kim, J.T. Kim, G.S. Lee, J.G. Kim, S.O. Kim, Tungsten nitride-coated graphene fibers for high-performance wearable supercapacitors. Nanoscale 12 (39), 20239–20249 (2020). https://doi.org/10.1039/d0nr06636b
J. Wang, K. Ma, J. Zhang, F. Liu, J. Cheng, Template-free synthesis of hierarchical hollow NiSx microspheres for supercapacitor. J. Colloid Interface Sci. 507 , 290–299 (2017). https://doi.org/10.1016/j.jcis.2017.07.095
Q. Zhang, L. Mei, X. Cao, Y. Tang, Z. Zeng, Intercalation and exfoliation chemistries of transition metal dichalcogenides. J. Mater. Chem. A 8 (31), 15417–15444 (2020). https://doi.org/10.1039/D0TA03727C
H. Tong, W. Bai, S. Yue, Z. Gao, L. Lu, L. Shen, S. Dong, J. Zhu, J. He, X. Zhang, Zinc cobalt sulfide nanosheets grown on nitrogen-doped graphene/carbon nanotube film as a high-performance electrode for supercapacitors. J. Mater. Chem. A 4 (29), 11256–11263 (2016). https://doi.org/10.1039/C6TA02249A
P. Kulkarni, S. Nataraj, R.G. Balakrishna, D. Nagaraju, M. Reddy, Nanostructured binary and ternary metal sulfides: synthesis methods and their application in energy conversion and storage devices. J. Mater. Chem. A 5 (42), 22040–22094 (2017). https://doi.org/10.1039/C7TA07329A
T. Li, Y. Bai, Y. Wang, H. Xu, H. Jin, Advances in transition-metal (Zn, Mn, Cu)-based MOFs and their derivatives for anode of lithium-ion batteries. Coord. Chem. Rev. 410 , 213221 (2020). https://doi.org/10.1016/j.ccr.2020.213221
Z. Zhang, Z. Huang, L. Ren, Y. Shen, X. Qi, J. Zhong, One-pot synthesis of hierarchically nanostructured Ni3S2 dendrites as active materials for supercapacitors. Electrochim. Acta 149 , 316–323 (2014). https://doi.org/10.1016/j.electacta.2014.10.097
D. Kim, P. Karthick Kannan, S. Mateti, C.-H. Chung, Indirect nanoconstruction morphology of Ni3S2 electrodes renovates the performance for electrochemical energy storage. ACS Appl. Energy Mater. 1 (12), 6945–6952 (2018). https://doi.org/10.1021/acsaem.8b01310
T. Chen, Z. Liu, Z. Liu, X. Tao, H. Fan, L. Guo, Fabrication of interconnected 2D/3D NiS/Ni3S4 composites for high performance supercapacitor. Mater. Lett. 248 , 1–4 (2019). https://doi.org/10.1016/j.matlet.2019.03.125
S. Liu, K. San Hui, K.N. Hui, J.M. Yun, K.H. Kim, Vertically stacked bilayer CuCo2O4/MnCo 2O4 heterostructures on functionalized graphite paper for high-performance electrochemical capacitors. J. Mater. Chem. A 4 (21), 8061–8071 (2016). https://doi.org/10.1039/C6TA00960C
J. Ren, Q. Meng, Z. Xu, X. Zhang, J. Chen, CoS2 hollow nanocubes derived from Co-Co Prussian blue analogue: High-performance electrode materials for supercapacitors. J. Electroanal. Chem. 836 , 30–37 (2019). https://doi.org/10.1016/j.jelechem.2019.01.049
H. Jia, Z. Wang, X. Zheng, Y. Cai, J. Lin, H. Liang, J. Qi, J. Cao, J. Feng, W. Fei, Controlled synthesis of MOF-derived quadruple-shelled CoS2 hollow dodecahedrons as enhanced electrodes for supercapacitors. Electrochim. Acta 312 , 54–61 (2019). https://doi.org/10.1016/j.electacta.2019.04.192
Z. Sun, X. Yang, H. Lin, F. Zhang, Q. Wang, F. Qu, Bifunctional iron disulfide nanoellipsoids for high energy density supercapacitor and electrocatalytic oxygen evolution applications. Inorg. Chem. Front. 6 (3), 659–670 (2019). https://doi.org/10.1039/C8QI01230J
D. Li, S. Song, J. Lu, J. Liang, Y. Zhang, L. Li, A general self-template-etched solution route for the synthesis of 2D γ-manganese sulfide nanoplates and their enhanced supercapacitive performance. New J. Chem. 43 (12), 4674–4680 (2019). https://doi.org/10.1039/C8NJ06143B
R.K. Mishra, G.W. Baek, K. Kim, H.-I. Kwon, S.H. Jin, One-step solvothermal synthesis of carnation flower-like SnS2 as superior electrodes for supercapacitor applications. Appl. Surf. Sci. 425 , 923–931 (2017). https://doi.org/10.1016/j.apsusc.2017.07.045
N. Parveen, S.A. Ansari, H.R. Alamri, M.O. Ansari, Z. Khan, M.H. Cho, Facile synthesis of SnS2 nanostructures with different morphologies for high-performance supercapacitor applications. ACS Omega 3 (2), 1581–1588 (2018). https://doi.org/10.1021/acsomega.7b01939
X.Y. Yu, X.W. David Lou, Mixed metal sulfides for electrochemical energy storage and conversion. Adv. Energy Mater. 8 (3), 1701–592 (2018). https://doi.org/10.1002/aenm.201701592
R. Xu, J. Lin, J. Wu, M. Huang, L. Fan, X. He, Y. Wang, Z. Xu, A two-step hydrothermal synthesis approach to synthesize NiCo2S4/NiS hollow nanospheres for high-performance asymmetric supercapacitors. Appl. Surf. Sci. 422 , 597–606 (2017). https://doi.org/10.1016/j.apsusc.2017.06.003
B.Y. Guan, L. Yu, X. Wang, S. Song, X.W.D. Lou, Formation of onion-like NiCo2S4 particles via sequential ion-exchange for hybrid supercapacitors. Adv. Mater. 29 (6), 1605051 (2017). https://doi.org/10.1002/adma.201605051
M. Govindasamy, S. Shanthi, E. Elaiyappillai, S.-F. Wang, P.M. Johnson, H. Ikeda, Y. Hayakawa, S. Ponnusamy, C. Muthamizhchelvan, Fabrication of hierarchical NiCo2S4@ CoS2 nanostructures on highly conductive flexible carbon cloth substrate as a hybrid electrode material for supercapacitors with enhanced electrochemical performance. Electrochim. Acta 293 , 328–337 (2019). https://doi.org/10.1016/j.electacta.2018.10.051
S. Yu, V.M.H. Ng, F. Wang, Z. Xiao, C. Li, L.B. Kong, W. Que, K. Zhou, Synthesis and application of iron-based nanomaterials as anodes of lithium-ion batteries and supercapacitors. J. Mater. Chem. A 6 (20), 9332–9367 (2018)
B. Balakrishnan, S.K. Balasingam, K. SivalingamNallathambi, A. Ramadoss, M. Kundu, J.S. Bak, I.H. Cho, P. Kandasamy, Y. Jun, H.-J. Kim, Facile synthesis of pristine FeS2 microflowers and hybrid rGO-FeS2 microsphere electrode materials for high performance symmetric capacitors. J. Ind. Eng. Chem. 71 , 191–200 (2019). https://doi.org/10.1016/j.jiec.2018.11.022
A.M. Zardkhoshoui, S.S.H. Davarani, A.A. Asgharinezhad, Designing graphene-wrapped NiCo2Se4 microspheres with petal-like FeS2 toward flexible asymmetric all-solid-state supercapacitors. Dalton Trans. 48 (13), 4274–4282 (2019). https://doi.org/10.1039/C9DT00009G
P. Naveenkumar, G.P. Kalaignan, Electrodeposited MnS on graphene wrapped Ni-Foam for enhanced supercapacitor applications. Electrochim. Acta 289 , 437–447 (2018). https://doi.org/10.1016/j.electacta.2018.09.100
X. Xu, X. Zhang, Y. Zhao, Y. Hu, An efficient hybrid supercapacitor based on battery-type MnS/reduced graphene oxide and capacitor-type biomass derived activated carbon. J. Mater. Sci.: Mater. Electron. 29 (10), 8410–8420 (2018). https://doi.org/10.1007/s10854-018-8852-3
R. Barik, N. Devi, V.K. Perla, S.K. Ghosh, K. Mallick, Stannous sulfide nanoparticles for supercapacitor application. Appl. Surf. Sci. 472 , 112–117 (2019). https://doi.org/10.1016/j.apsusc.2018.03.172
J.N. Coleman, M. Lotya, A. O’Neill, S.D. Bergin, P.J. King, U. Khan, K. Young, A. Gaucher, S. De, R.J. Smith, Two-dimensional nanosheets produced by liquid exfoliation of layered materials. Science 331 (6017), 568–571 (2011). https://doi.org/10.1126/science.1194975
M. Sajjad, W. Lu, Covalent organic frameworks based nanomaterials: design, synthesis, and current status for supercapacitor applications: a review. J. Energy Storage 39 , 102618 (2021). https://doi.org/10.1016/j.est.2021.102618
N.S. Arul, J.I. Han, Facile hydrothermal synthesis of hexapod-like two dimensional dichalcogenide NiSe2 for supercapacitor. Mater. Lett. 181 , 345–349 (2016). https://doi.org/10.1016/j.matlet.2016.06.065
Y. Gu, W. Du, Y. Darrat, M. Saleh, Y. Huang, Z. Zhang, S. Wei, In situ growth of novel nickel diselenide nanoarrays with high specific capacity as the electrode material of flexible hybrid supercapacitors. Appl. Nanosci. 10 (5), 1591–1601 (2019). https://doi.org/10.1007/s13204-019-01234-8
P. Pazhamalai, K. Krishnamoorthy, S. Sahoo, S.J. Kim, Two-dimensional molybdenum diselenide nanosheets as a novel electrode material for symmetric supercapacitors using organic electrolyte. Electrochim. Acta 295 , 591–598 (2019). https://doi.org/10.1016/j.electacta.2018.10.191
Y. Gu, L.-Q. Fan, J.-L. Huang, C.-L. Geng, J.-M. Lin, M.-L. Huang, Y.-F. Huang, J.-H. Wu, N-doped reduced graphene oxide decorated NiSe2 nanoparticles for high-performance asymmetric supercapacitors. J. Power Sources 425 , 60–68 (2019). https://doi.org/10.1016/j.jpowsour.2019.03.123
A. Gopalakrishnan, S. Badhulika, Binder-free polyaniline sheathed crumpled cobalt diselenide nanoparticles as an advanced electrode for high specific energy asymmetric supercapacitor. J. Energy Storage 41 , 102853 (2021). https://doi.org/10.1016/j.est.2021.102853
C. Miao, X. Yin, G. Xia, K. Zhu, K. Ye, Q. Wang, J. Yan, D. Cao, G. Wang, Facile microwave-assisted synthesis of cobalt diselenide/reduced graphene oxide composite for high-performance supercapacitors. Appl. Surf. Sci. 543 , 148811 (2021). https://doi.org/10.1016/j.apsusc.2020.148811
C. Miao, X. Xiao, Y. Gong, K. Zhu, K. Cheng, K. Ye, J. Yan, D. Cao, G. Wang, P. Xu, Facile synthesis of metal-organic framework-derived CoSe2 nanoparticles embedded in the N-doped carbon nanosheet array and application for supercapacitors. ACS Appl. Mater. Interfaces. 12 (8), 9365–9375 (2020). https://doi.org/10.1021/acsami.9b22606
C.V.V.M. Gopi, A.E. Reddy, J.-S. Bak, I.-H. Cho, H.-J. Kim, One-pot hydrothermal synthesis of tungsten diselenide/reduced graphene oxide composite as advanced electrode materials for supercapacitors. Mater. Lett. 223 , 57–60 (2018). https://doi.org/10.1016/j.matlet.2018.04.023
S.R. Marri, S. Ratha, C.S. Rout, J. Behera, 3D cuboidal vanadium diselenide embedded reduced graphene oxide hybrid structures with enhanced supercapacitor properties. Chem. Commun. 53 (1), 228–231 (2017). https://doi.org/10.1039/C6CC08035A
H.M. El Sharkawy, D.M. Sayed, A.S. Dhmees, R.M. Aboushahba, N.K. Allam, Facile synthesis of nanostructured binary Ni–Cu phosphides as advanced battery materials for asymmetric electrochemical supercapacitors. ACS Appl. Energy Mater. 3 (9), 9305–9314 (2020). https://doi.org/10.1021/acsaem.0c01630
X. Li, A.M. Elshahawy, C. Guan, J. Wang, Metal phosphides and phosphates-based electrodes for electrochemical supercapacitors. Small 13 (39), 1701530 (2017). https://doi.org/10.1002/smll.201701530
J. Theerthagiri, A.P. Murthy, S.J. Lee, K. Karuppasamy, S.R. Arumugam, Y. Yu, M.M. Hanafiah, H.-S. Kim, V. Mittal, M.Y. Choi, Recent progress on synthetic strategies and applications of transition metal phosphides in energy storage and conversion. Ceram. Int. 47 (4), 4404–4425 (2021). https://doi.org/10.1016/j.ceramint.2020.10.098
Y.-C. Chen, Z.-B. Chen, Y.-G. Lin, Y.-K. Hsu, Synthesis of copper phosphide nanotube arrays as electrodes for asymmetric supercapacitors. ACS Sustain. Chem. Eng. 5 (5), 3863–3870 (2017). https://doi.org/10.1021/acssuschemeng.6b03006
F. Liang, L. Huang, L. Tian, J. Li, H. Zhang, S. Zhang, Microwave-assisted hydrothermal synthesis of cobalt phosphide nanostructures for advanced supercapacitor electrodes. CrystEngComm 20 (17), 2413–2420 (2018). https://doi.org/10.1039/c8ce00054a
Z. Zheng, M. Retana, X. Hu, R. Luna, Y.H. Ikuhara, W. Zhou, Three-dimensional cobalt phosphide nanowire arrays as negative electrode material for flexible solid-state asymmetric supercapacitors. ACS Appl. Mater. Interfaces. 9 (20), 16986–16994 (2017). https://doi.org/10.1021/acsami.7b01109
G. Zhu, L. Yang, W. Wang, M. Ma, J. Zhang, H. Wen, D. Zheng, Y. Yao, Hierarchical three-dimensional manganese doped cobalt phosphide nanowire decorated nanosheet cluster arrays for high-performance electrochemical pseudocapacitor electrodes. Chem. Commun. 54 (66), 9234–9237 (2018). https://doi.org/10.1039/C8CC02475H
MSP, S., G. Gnanasekaran, P. Pazhamalai, S. Sahoo, M.M. Hossain, R.M. Bhattarai, S.-J. Kim, and Y.S. Mok, Hierarchically porous nanostructured nickel phosphide with carbon particles embedded by dielectric barrier discharge plasma deposition as a binder-free electrode for hybrid supercapacitors. ACS Sustainable Chemistry & Engineering, 2019. 7 (17): p. 14805–14814. Doi: https://doi.org/10.1021/acssuschemeng.9b02832 .
P. Sivakumar, M.G. Jung, C.J. Raj, H.H. Rana, H.S. Park, 1D interconnected porous binary transition metal phosphide nanowires for high performance hybrid supercapacitors. Int. J. Energy Res. (2021). https://doi.org/10.1002/er.6874
S. Li, M. Hua, Y. Yang, W. Huang, X. Lin, L. Ci, J. Lou, P. Si, Self-supported multidimensional Ni–Fe phosphide networks with holey nanosheets for high-performance all-solid-state supercapacitors. J. Mater. Chem. A 7 (29), 17386–17399 (2019). https://doi.org/10.1039/C9TA04832D
T.T. Nguyen, J. Balamurugan, N.H. Kim, J.H. Lee, Hierarchical 3D Zn–Ni–P nanosheet arrays as an advanced electrode for high-performance all-solid-state asymmetric supercapacitors. J. Mater. Chem. A 6 (18), 8669–8681 (2018). https://doi.org/10.1039/C8TA01184B
A.A. Saleh, A. Amer, D.M. Sayed, N.K. Allam, A facile electrosynthesis approach of Mn-Ni-Co ternary phosphides as binder-free active electrode materials for high-performance electrochemical supercapacitors. Electrochim. Acta 380 , 138197 (2021). https://doi.org/10.1016/j.electacta.2021.138197
D. Ling, N. Lee, T. Hyeon, Chemical synthesis and assembly of uniformly sized iron oxide nanoparticles for medical applications. Acc. Chem. Res. 48 (5), 1276–1285 (2015). https://doi.org/10.1021/acs.accounts.5b00038
S. Bai, H. Zou, H. Dietsch, Y.C. Simon, C. Weder, Functional iron oxide nanoparticles as reversible crosslinks for magnetically addressable shape-memory polymers. Macromol. Chem. Phys. 215 (5), 398–404 (2014). https://doi.org/10.1002/macp.201300632
B. Šljukić, C.E. Banks, R.G. Compton, Iron oxide particles are the active sites for hydrogen peroxide sensing at multiwalled carbon nanotube modified electrodes. Nano Lett. 6 (7), 1556–1558 (2006). https://doi.org/10.1021/nl060366v
G. Li, R. Li, W. Zhou, A wire-shaped supercapacitor in micrometer size based on Fe3O4 nanosheet arrays on Fe wire. Nano-Micro Lett. 9 (4), 1–8 (2017). https://doi.org/10.1007/s40820-017-0147-3
T. Valdés-Solís, P. Valle-Vigón, S. Álvarez, G. Marbán, A.B. Fuertes, Manganese ferrite nanoparticles synthesized through a nanocasting route as a highly active Fenton catalyst. Catal. Commun. 8 (12), 2037–2042 (2007). https://doi.org/10.1016/j.catcom.2007.03.030
E. Peng, E.S.G. Choo, P. Chandrasekharan, C.T. Yang, J. Ding, K.H. Chuang, J.M. Xue, Synthesis of manganese ferrite/graphene oxide nanocomposites for biomedical applications. Small 8 (23), 3620–3630 (2012). https://doi.org/10.1002/smll.201201427
Z. Li, S.X. Wang, Q. Sun, H.L. Zhao, H. Lei, M.B. Lan, Z.X. Cheng, X.L. Wang, S.X. Dou, G.Q. Lu, Ultrasmall manganese ferrite nanoparticles as positive contrast agent for magnetic resonance imaging. Adv. Healthcare Mater. 2 (7), 958–964 (2013). https://doi.org/10.1002/adhm.201200340
M.S. Amulya, H. Nagaswarupa, M.A. Kumar, C. Ravikumar, S. Prashantha, K. Kusuma, Sonochemical synthesis of NiFe2O4 nanoparticles: Characterization and their photocatalytic and electrochemical applications. Appl. Surf. Sci. Adv. 1 , 100023 (2020). https://doi.org/10.1016/j.apsadv.2020.100023
S. Sharifi, A. Yazdani, K. Rahimi, Incremental substitution of Ni with Mn in NiFe2O4 to largely enhance its supercapacitance properties. Sci. Rep. 10 (1), 10916 (2020). https://doi.org/10.1038/s41598-020-67802-z
B. Bhujun, M.T. Tan, A.S. Shanmugam, Study of mixed ternary transition metal ferrites as potential electrodes for supercapacitor applications. Results Phys. 7 , 345–353 (2017). https://doi.org/10.1016/j.rinp.2016.04.010
A.A. Tahir, H.A. Burch, K.U. Wijayantha, B.G. Pollet, A new route to control texture of materials: Nanostructured ZnFe2O4 photoelectrodes. Int. J. Hydrogen Energy 38 (11), 4315–4323 (2013). https://doi.org/10.1016/j.ijhydene.2013.01.130
A.A. Tahir, K.U. Wijayantha, Photoelectrochemical water splitting at nanostructured ZnFe2O4 electrodes. J. Photochem. Photobiol. A 216 (2–3), 119–125 (2010). https://doi.org/10.1016/j.jphotochem.2010.07.032
N.K. Sahu, J. Gupta, D. Bahadur, PEGylated FePt–Fe 3 O 4 composite nanoassemblies (CNAs): in vitro hyperthermia, drug delivery and generation of reactive oxygen species (ROS). Dalton Trans. 44 (19), 9103–9113 (2015). https://doi.org/10.1039/C4DT03470H
S.S. Raut, B.R. Sankapal, First report on synthesis of ZnFe2O4 thin film using successive ionic layer adsorption and reaction: approach towards solid-state symmetric supercapacitor device. Electrochim. Acta 198 , 203–211 (2016). https://doi.org/10.1016/j.electacta.2016.03.059
V. Blanco-Gutierrez, R. Saez-Puche, M.J. Torralvo-Fernandez, Superparamagnetism and interparticle interactions in ZnFe2O4 nanocrystals. J. Mater. Chem. 22 (7), 2992–3003 (2012). https://doi.org/10.1039/C1JM14856G
P. Guo, G. Zhang, J. Yu, H. Li, X. Zhao, Controlled synthesis, magnetic and photocatalytic properties of hollow spheres and colloidal nanocrystal clusters of manganese ferrite. Colloids Surf., A 395 , 168–174 (2012). https://doi.org/10.1016/j.colsurfa.2011.12.027
J. Wang, Q. Chen, B. Hou, Z. Peng, Synthesis and magnetic properties of single-crystals of MnFe2O4 nanorods. Eur. J. Inorg. Chem. 2004 (6), 1165–1168 (2004). https://doi.org/10.1002/ejic.200300555
H.-M. Fan, J.-B. Yi, Y. Yang, K.-W. Kho, H.-R. Tan, Z.-X. Shen, J. Ding, X.-W. Sun, M.C. Olivo, Y.-P. Feng, Single-crystalline MFe2O4 nanotubes/nanorings synthesized by thermal transformation process for biological applications. ACS Nano 3 (9), 2798–2808 (2009). https://doi.org/10.1021/nn9006797
L. Cui, P. Guo, G. Zhang, Q. Li, R. Wang, M. Zhou, L. Ran, X. Zhao, Facile synthesis of cobalt ferrite submicrospheres with tunable magnetic and electrocatalytic properties. Colloids Surf., A 423 , 170–177 (2013). https://doi.org/10.1016/j.colsurfa.2013.01.064
N. Bao, L. Shen, Y.-H.A. Wang, J. Ma, D. Mazumdar, A. Gupta, Controlled growth of monodisperse self-supported superparamagnetic nanostructures of spherical and rod-like CoFe2O4 nanocrystals. J. Am. Chem. Soc. 131 (36), 12900–12901 (2009). https://doi.org/10.1021/ja905811h
Y. Xu, J. Wei, J. Yao, J. Fu, D. Xue, Synthesis of CoFe2O4 nanotube arrays through an improved sol–gel template approach. Mater. Lett. 62 (8–9), 1403–1405 (2008). https://doi.org/10.1016/j.matlet.2007.08.066
S. Zhang, D. Dong, Y. Sui, Z. Liu, H. Wang, Z. Qian, W. Su, Preparation of core shell particles consisting of cobalt ferrite and silica by sol–gel process. J. Alloy Compd. 415 (1–2), 257–260 (2006). https://doi.org/10.1016/j.jallcom.2005.07.048
H. Aijun, L. Juanjuan, Y. Mingquan, L. Yan, P. Xinhua, Preparation of nano-MnFe2O4 and its catalytic performance of thermal decomposition of ammonium perchlorate. Chin. J. Chem. Eng. 19 (6), 1047–1051 (2011). https://doi.org/10.1016/S1004-9541(11)60090-6
S. Sartale, C. Lokhande, A room temperature two-step electrochemical process for large area nanocrystalline ferrite thin films deposition. J. Electroceram. 15 (1), 35–44 (2005). https://doi.org/10.1007/s10832-005-1076-y
V. Vignesh, K. Subramani, M. Sathish, R. Navamathavan, Electrochemical investigation of manganese ferrites prepared via a facile synthesis route for supercapacitor applications. Colloids Surf., A 538 , 668–677 (2018). https://doi.org/10.1016/j.colsurfa.2017.11.045
R. Roshani, A. Tadjarodi, Synthesis of ZnFe2O4 nanoparticles with high specific surface area for high-performance supercapacitor. J. Mater. Sci.: Mater. Electron. 31 (24), 23025–23036 (2020). https://doi.org/10.1007/s10854-020-04830-5
B. Saravanakumar, S. Ramachandran, G. Ravi, V. Ganesh, R.K. Guduru, R. Yuvakkumar, Electrochemical performances of monodispersed spherical CuFe2O4 nanoparticles for pseudocapacitive applications. Vacuum 168 , 108798 (2019). https://doi.org/10.1016/j.vacuum.2019.108798
H. Kennaz, A. Harat, O. Guellati, D.Y. Momodu, F. Barzegar, J.K. Dangbegnon, N. Manyala, M. Guerioune, Synthesis and electrochemical investigation of spinel cobalt ferrite magnetic nanoparticles for supercapacitor application. J. Solid State Electrochem. 22 (3), 835–847 (2017). https://doi.org/10.1007/s10008-017-3813-y
G. Nabi, W. Raza, M.A. Kamran, T. Alharbi, M. Rafique, M.B. Tahir, S. Hussain, N. Khalid, N. Malik, R.S. Ahmed, Role of cerium-doping in CoFe2O4 electrodes for high performance supercapacitors. J. Energy Storage 29 , 101452 (2020). https://doi.org/10.1016/j.est.2020.101452
M. Sethi, U.S. Shenoy, S. Muthu, D.K. Bhat, Facile solvothermal synthesis of NiFe 2 O 4 nanoparticles for high-performance supercapacitor applications. Front. Mater. Sci. 14 (2), 120–132 (2020). https://doi.org/10.1007/s11706-020-0499-3
M. Khairy, W. Bayoumy, S. Selima, M. Mousa, Studies on characterization, magnetic and electrochemical properties of nano-size pure and mixed ternary transition metal ferrites prepared by the auto-combustion method. J. Mater. Res. 35 (20), 2652–2663 (2020). https://doi.org/10.1557/jmr.2020.200
B. Nawaz, G. Ali, M.O. Ullah, S. Rehman, F. Abbas, Investigation of the electrochemical properties of Ni0.5Zn0.5Fe2O4 as binder-based and binder-free electrodes of supercapacitors. Energies 14 (11), 3297 (2021). https://doi.org/10.3390/en14113297
Download references
Author information
Authors and affiliations.
Department of Physics and Engineering Physics, Central Connecticut State University, New Britain, CT, 06050, USA
Rahul Singhal
Department of Physics, Chaudhary Charan Singh University, Meerut, 250004, India
Manika Chaudhary, Shrestha Tyagi, Deepanshi Tyagi, Vanshika Bhardwaj & Beer Pal Singh
You can also search for this author in PubMed Google Scholar
Contributions
All authors have equal contribution.
Corresponding authors
Correspondence to Rahul Singhal or Beer Pal Singh .
Ethics declarations
Conflict of interest.
The authors declare no conflicts of interest.
Data availability
The data/findings reported in this review article is available in respective references and doi is given for all references in this article.
Rights and permissions
Reprints and permissions
About this article
Singhal, R., Chaudhary, M., Tyagi, S. et al. Recent developments in transition metal-based nanomaterials for supercapacitor applications. Journal of Materials Research 37 , 2124–2149 (2022). https://doi.org/10.1557/s43578-022-00598-y
Download citation
Received : 18 February 2022
Accepted : 09 May 2022
Published : 02 June 2022
Issue Date : 14 July 2022
DOI : https://doi.org/10.1557/s43578-022-00598-y
Share this article
Anyone you share the following link with will be able to read this content:
Sorry, a shareable link is not currently available for this article.
Provided by the Springer Nature SharedIt content-sharing initiative
- Supercapacitor
- Transition metals
- Specific capacitance
- Nanomaterials
- Scanning electron microscopy
- Charge–discharge
- Find a journal
- Publish with us
- Track your research

Maintenance work is planned for Wednesday 1st May 2024 from 9:00am to 11:00am (BST).
During this time, the performance of our website may be affected - searches may run slowly and some pages may be temporarily unavailable. If this happens, please try refreshing your web browser or try waiting two to three minutes before trying again.
We apologise for any inconvenience this might cause and thank you for your patience.
Chemical Society Reviews
The mechanism of water oxidation using transition metal-based heterogeneous electrocatalysts.

* Corresponding authors
a Key Laboratory of Applied Surface and Colloid Chemistry, Ministry of Education, School of Chemistry and Chemical Engineering, Shaanxi Normal University, Xi’an 710119, P. R. China E-mail: [email protected] , [email protected] , [email protected]
The water oxidation reaction, a crucial process for solar energy conversion, has garnered significant research attention. Achieving efficient energy conversion requires the development of cost-effective and durable water oxidation catalysts. To design effective catalysts, it is essential to have a fundamental understanding of the reaction mechanisms. This review presents a comprehensive overview of recent advancements in the understanding of the mechanisms of water oxidation using transition metal-based heterogeneous electrocatalysts, including Mn, Fe, Co, Ni, and Cu-based catalysts. It highlights the catalytic mechanisms of different transition metals and emphasizes the importance of monitoring of key intermediates to explore the reaction pathway. In addition, advanced techniques for physical characterization of water oxidation intermediates are also introduced, for the purpose of providing information for establishing reliable methodologies in water oxidation research. The study of transition metal-based water oxidation electrocatalysts is instrumental in providing novel insights into understanding both natural and artificial energy conversion processes.
Article information
Download citation, permissions.

S. Yang, X. Liu, S. Li, W. Yuan, L. Yang, T. Wang, H. Zheng, R. Cao and W. Zhang, Chem. Soc. Rev. , 2024, Advance Article , DOI: 10.1039/D3CS01031G
To request permission to reproduce material from this article, please go to the Copyright Clearance Center request page .
If you are an author contributing to an RSC publication, you do not need to request permission provided correct acknowledgement is given.
If you are the author of this article, you do not need to request permission to reproduce figures and diagrams provided correct acknowledgement is given. If you want to reproduce the whole article in a third-party publication (excluding your thesis/dissertation for which permission is not required) please go to the Copyright Clearance Center request page .
Read more about how to correctly acknowledge RSC content .
Social activity
Search articles by author.
This article has not yet been cited.
Advertisements
- Open access
- Published: 24 February 2021
Synthesis, characterization, and biological studies of some biometal complexes
- Vinay Kumar Srivastava 1
Future Journal of Pharmaceutical Sciences volume 7 , Article number: 51 ( 2021 ) Cite this article
2615 Accesses
6 Citations
Metrics details
Metal complexes Cu[C 13 H 8 O 4 N] 2 2, Ni[C l3 H 8 O 4 N] 2 3, and Co[C 13 H 8 O 4 N] 2 4 of bioinorganic relevance have been synthesized with the Schiff base ligand 2-furylglyoxal–anthranilic acid (FGAA) [C 13 H 9 O 4 N] 1 .
All the complexes are well characterized by various spectral and physical methods. The antimicrobial activity of the complexes has been studied against some of the pathogenic bacteria and fungi.
Results indicate that complexes have higher antimicrobial activity than the free ligand. This would suggest that chelation reduces considerably the polarity of the metal ions in the complexes which in turn increases the hydrophobic character of the chelate and thus enables permeation, through the lipid layer of microorganisms. All the complexes were assessed for their anticancer studies against a panel of selected cancer cells HOP62 and BT474 respectively. Results showed that the complexes are promising chemotherapeutic alternatives in the search of anticancer agents. The fluorescence quenching phenomenon is observed in the Schiff base metal complexes.
The octahedral transition metal complexes 2, 3, and 4 have been obtained by treatment of ligand 2-furylglyoxal-anthranilic acid (FGAA) 1 with metal acetate. Complexes under investigations have shown antimicrobial, potential anticancer, and the DNA binding studies.
Graphical abstract
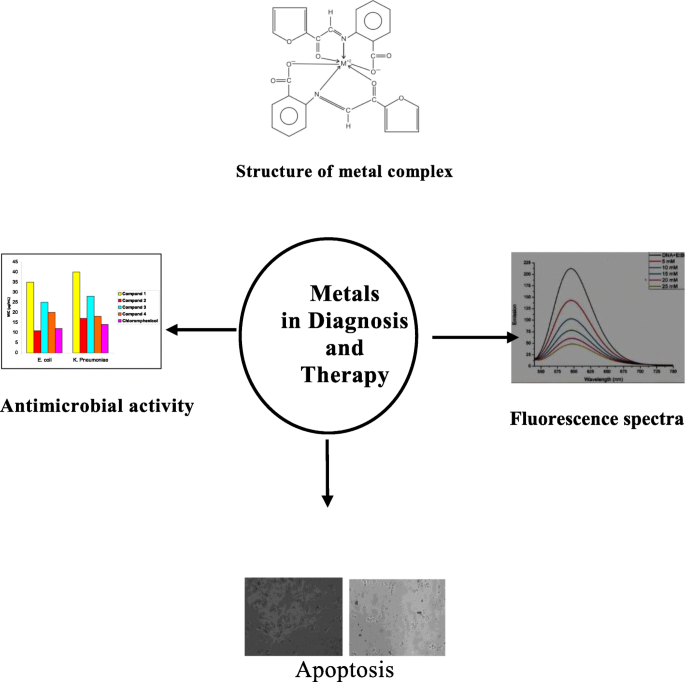
The chemistry of transition metal complexes has received considerable attention largely due to their catalytic and bioinorganic relevance. Such complexes are also important due to their potential biological activities such as antibacterial, antifungal, antimalarial, and antitumor [ 1 , 2 , 3 , 4 ]. Medicinal inorganic chemistry is comparatively a new discipline which developed after the serendipitous discovery of the antitumor activity of cisplatin [ 5 , 6 , 7 ]. The clinical success of this platinum complex has stimulated considerable interest in the search for new metal complexes as modern therapeutics, diagnostic, and radiopharmaceutical agents. Copper, nickel, and cobalt complexes are used in the treatment of many diseases including cancer and as potential hypoxia-activated prodrugs [ 8 , 9 , 10 , 11 , 12 , 13 , 14 ]. Coordination compounds which form coordinate bonds via the sulfur, oxygen, and nitrogen donor atoms are well known and have a long history. The interest in preparation of new metal complexes gained the tendency of studying the interactions of metal complexes with DNA for their applications in biotechnology and medicine.
Deoxyribonucleic acid (DNA) is the primary target molecule for most anticancer and antiviral therapies according to cell biologists. Investigation on the interaction of DNA with small molecules is important in the design of new type of pharmaceutical molecule. Schiff base constitutes an important class of nitrogen donor ligands and occupy a prominent position among the recent achievement in the field of coordination chemistry. The azomethine which is the functional group of Schiff base is aided in forming a stable complex. The chemistry of Schiff base metal complexes is exploited in industries, technologies, and in medicinal fields. The present investigations deal with the synthesis, characterization, antimicrobial, anticancer, and DNA cleavage studies of Cu(II), Ni(II), and Co(II) metal complexes containing Schiff base ligand 2-furylglyoxal-anthranilic acid (FGAA).
All reagents used were of analytical grade and used as purchased commercially; however, the solvents were purified by the standard procedure [ 15 ]. The ligand 2-furylglyoxal-anthranilic acid (FGAA) was prepared by the reported procedure [ 16 , 17 , 18 , 19 , 20 ]. C, H, and N were analyzed on Carlo-Erba microanalyzer. Metal contents were estimated by standard procedure [ 21 ]. FTIR was recorded on Thermo Nicolet Avater 370. Electronic spectra on Shimadzu UV-160A spectrophotometer. The conductance measurements were carried out on a metal CM-180 Eliodigital conductivity meter. Magnetic studies were done by a Guoy balance using Hg [Co (SCN) 4 ] as the calibrant.
1 H and 13 C NMR spectra in dimethyl sulfoxide (DMSO) were recorded on a Brucker WH 300 (200 MHz) and Varian Gemini (200 MHz) spectrometers using tetramethylsilane (TMS) as an internal reference.
The in vitro antimicrobial screening effects of the investigated compounds were tested against the bacterial species: Escherichia coli , ( E. coli ) and Klbsiella pneumoniae ( K. pneumoniae ), and fungal species: Aspergillus niger ( A. niger ) and Candida albicans ( C. albicans ) by using Kirby Bauer Disk diffusion method [ 22 , 23 , 24 ]. Chloramphenicol and nystatin were used as the standard antibacterial and antifungal agents. The tested compounds were dissolved in DMF solution (which has no inhibition activity) and solution soaked in filter paper disk of 5 mm diameter and 1 mm thickness. The disks were incubated 24 h for bacterial and 72 h for fungal species at 37 °C. The minimum inhibitory concentration (MIC) value of the compounds was determined by the serial dilution method [ 25 , 26 , 27 ].
The in vitro cancer studies of all the compounds were assessed for their anti-proliferation test against a panel of selected human cancer cell lines such as HOP62 (lung) and BT 474 (breast) by using SRB (sulforhodamine B) assay [ 28 , 29 , 30 , 31 , 32 , 33 , 34 , 35 , 36 , 37 ] concentration of drug used 10, 20, 40, and 80 μg/mL ADR (adrimycin) was used as a positive control which controls cells with definite structure and clear cell wall without degeneration. Each drug was assayed inducing 50% growth inhibition (GI 50 ), total growth inhibition (TGI), and 50% cytotoxicity (LC 50 ) after a 48 h incubation period were calculated by linear interpolation from the observed data points. Fluorescence measurements were recorded on an F-7000 FL spectrophotometer at room temperature.
Synthesis of metal complexes (2-4)
Metal complexes of Cu[C 13 H 8 O 4 N) 2 2, Ni [C 13 H 8 O 4 N] 2 3 , and Co [C 13 H 8 O 4 N] 2 4, were synthesized by the addition of ethanolic solution of ligand 2-furylglyoxal–anthranilic acid 1 (2 mmol) copper acetate/nickel acetate/cobalt acetate (1 mmol). The mixture was magnetically stirred and refluxed for 2 h. The complexes obtained were filtered, washed with ethanol, and dried.
All the metal complexes were colored, non-hygroscopic in nature, and stable at room temp. They were insoluble in common organic solvents but soluble in DMF and DMSO. The results of the elemental analysis are in good agreement with the calculated values. The molar conductance value indicates their non-electrolytic nature. Physical and analytical data of complexes are summarized in Table 1 .
On the basis of analytical and spectral data, octahedral geometry has been assigned to the complexes. The results of antimicrobial activity and anticancer studies indicate metal complexes are much more active as compared to ligand fragments. Fluorescence quenching phenomena are observed in its metal complexes by fluorescence studies.
IR spectral studies
Infrared spectra of free ligand, a sharp band [ 38 , 39 , 40 ] appeared at 1615-1590 cm –1 ascribed to the stretching vibrations of azomethine group and was shifted to lower frequency region after complexation suggesting thereby the participation of imine nitrogen. A strong band appeared at 1735-1690 cm –1 in the IR spectra of ligand (FGAA) which is due to the presence of stretching vibration of carbonyl group coordination through this carbonyl oxygen to the central metal ion is confirmed by a negative shift in this frequency in the spectra of corresponding metal complexes. IR spectra of ligand displays a bond of medium intensity in the region of 3550-3490 cm –1 due to the –OH stretching vibration of free –CO 2 H group. Coordination of ligand as a consequence of deprotonation of – CO 2 H group is evident by the disappearance of the above band in the IR spectra of respective complexes [ 41 , 42 ]. Furthermore, the asymmetrical and symmetrical vibrations of COO – group appeared at 1560-1535 cm –1 and 1340-1325 cm –1 Δν (as–s) value 220-210 cm –1 further indicate the coordination through unidentate carboxylate group. Some new bands appeared in the IR spectra of metal complexes at 550-530 cm –1 , 450-430 cm –1 , and 335-325 cm –1 are probably due to the formation of M–O, M–N, and M–S bonds respectively which further give additional evidences in favor of the coordination of metals through azomethine nitrogen, carbonyl oxygen, and carboxylate group.
Electronic spectral and magnetic studies
Divalent copper having a d 9 configuration give rise to a 2D free ion term which split into a regular octahedral environment into a lower doublet 2 E g and an upper triplet 2 T 2g levels. In electronic spectra [ 43 , 44 , 45 , 46 ] of a true octahedral system, only one band due to 2 E g → 2 T 2g transitions is expected but true octahedral structures are not common. Therefore, instead of a one broad band due to 2 E g → 2 T 2g transition. These transitions from the ground state 2 B 1g → 2 A 1g → 2 B 2g and 2 E g are expected as a consequence of John-Tellers configuration stability. The 2 E g orbitals separate so that one goes up as much as the other goes down.
The T 2g orbitals separate in such a way that the doubly degenerate pair goes down only half as far as the single orbital goes up therefore in case of Cu (II); there is no net energy change for T 2g electrons since four are stabilized while two are destabilized due to which Cu(II) complex shows distortion in an octahedral geometry. The electronic spectra of Cu(II) complex displays three spectral bands in the region 10635, 14850, 16345 cm –1 which are in good agreement with the distorted geometry of complex under investigation. This geometry is further supported by the magnetic moment value 1.98 B.M. of the complex. In the Ni(II) complex, three bands in the range 10750, 1665, 25650 cm –1 corresponding to the transition 3 A 2g → 3 T 2g → 3 T 1g and 3 T 1g (P) are observed which clearly indicate the octahedral geometry. The theoretical value of ν 2 /ν 1 for octahedral Ni(II) complex is found 1.55. The observed value lies 1.60 which is in conformity with the distorted octahedral geometry of the ligand around central Ni(II) ion lowering the ratio of ν 2 /ν 1 may be attributed due to configuration interaction between T 1g (P) and T 1g (F) excited state. The octahedral geometry is further supported by their magnetic value 3.14 B.M. In octahedrally surrounded Co(II) ions, three bands in the region 8000, 15616, 18175 cm –1 are expected which may be assigned to 4 T 1g to 4 T 2g (F) (ν 1 ), 4 A 2g (F) (ν 2 ), and 4 T 1g (P) (ν 3 ) transitions respectively. The 4 A 2g transition is very weak and often appears as shoulder. Cobalt complex possesses an octahedral geometry which is further confirmed by the energy ratio ν 2 /ν 1 lies 1.95 and magnetic moment value 4.60 B.M.
NMR spectral studies
In the 1 H NMR spectra of free Schiff base ligand, the signals were appeared in the range of 7.15-7.20 ppm due to (HC=N) proton [ 47 ]. However, in the spectra of Schiff base metal complexes of Cu(II), Ni(II), and Co(II), the signals were observed in the downfield regions of 8.0–9.0 ppm supporting the coordination of iminonitrogen atom to Cu (II)/Ni (II)/Co (II) [ 48 ] while the free ligand NMR spectra has a characteristic NMR signal for carboxyl group proton in the 10.5-12.5 ppm range, the disappearance of this signal in the 1 H NMR spectra of metal complexes indicating the involvement of carboxylate ion oxygen in chelation through deprotonation. There is no appreciable change in the peak position corresponding to NH and aromatic protons. The 13 C–NMR signals for the metal, complexes are assigned by the comparison with the spectra of corresponding free Schiff base ligand. A downfield shift of CH = N group in the range of 150-160 ppm and for 175-182.5 ppm. In the complexes, NMR spectra indicate that the ligand coordinates through both the nitrogen atom of CH = N and the oxygen of COO — ion [ 49 , 50 , 51 , 52 ] (Fig. 1 ).
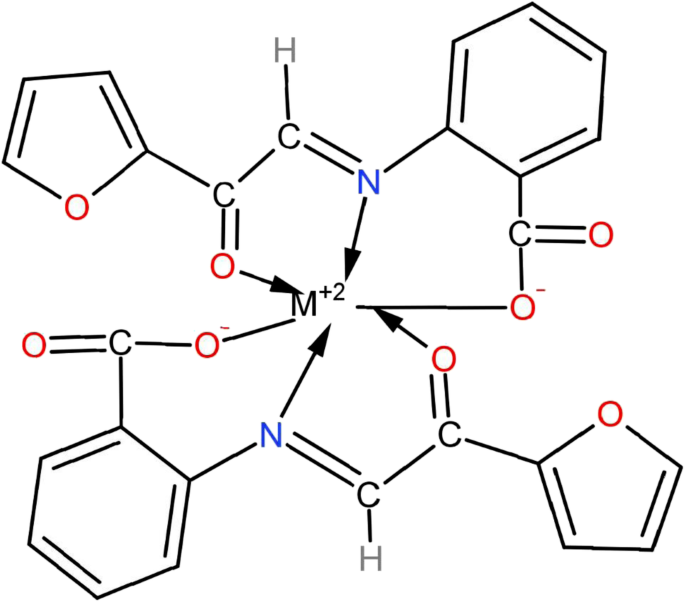
The proposed structure of metal complex M = Cu(II) or Ni(II) or Co(II)
In vitro antimicrobial studies
The antibacterial and antifungal activity of the ligand and complexes [ 53 , 54 ] were assayed against some of the bacteria and fungi. DMF is used as negative control and chloramphenicol is used as a positive standard for antibacterial and nystatin for antifungal activities (Fig. 2 a and b). The minimum inhibitory concentration (MIC) value of the compounds was determined by the serial dilution method and is given in Table 2 .
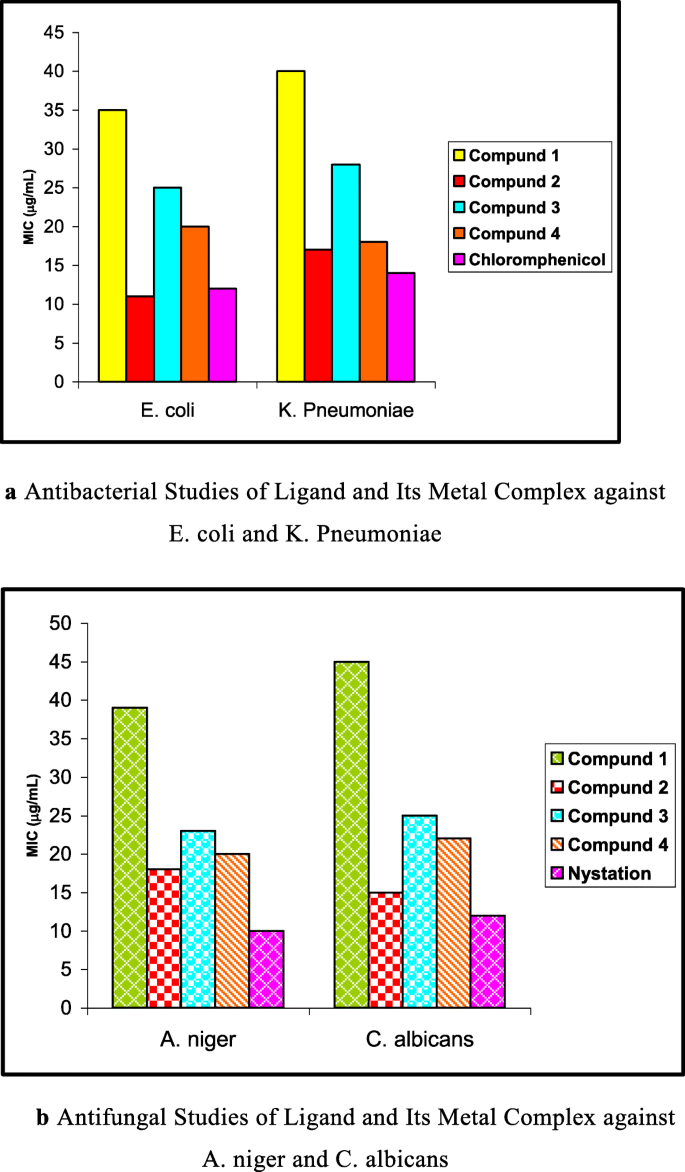
a Antibacterial studies of ligand and its metal complex against E. coli and K. pneumoniae . b Antifungal studies of ligand and its metal complex against A. niger and C. albicans
The in vitro antimicrobial activity results revealed that complexes are more microbial toxic than the ligand. The activity order of the synthesized complexes and ligand are as follows 2 > 4 > 3 > 1 .
Such increased activity of the metal chelates can be explained on the basis of Tweedy’s chelation theory on chelation, the polarity of the metal ion will be reduced to a greater extent due to the overlap of the ligand orbital and partial sharing of the positive charge of the metal ion with donor groups. Further, it increases the delocalization of π electrons over the whole chelate ring and enhances the penetration of the metal complexes into lipid membranes and blocking of the metal-binding sites in the enzymes of microogranism. These complexes also disturb the respiration process of the cell and thus block the synthesis of proteins which restricts further growth of the organism. The complex 2 shows higher antimicrobial activity than other complex 3 and 4 complex. The variation in the effectiveness of different compounds against different organisms depends either on the impermeability of the cells of the microbes or on differences in ribosome of microbial cells. Further, lipophilicity which controls the rate of entry of molecules into the cells is modified by coordination so compounds 2 , 3 , and 4 can become more active than compound 1 compared to the standard compounds chloramphenicol and nystatin (Fig. 2 a and b); the present metal complexes are much less active against the representative strains of microorganism [ 55 , 56 ].
In vitro anticancer studies
The data obtained by the SRB assay show that metal complexes 2 and 3 have inhibitory effects on the growth of HOP62 (Tables 3 and 4 ) (Fig. 3 ) and BT474 (Tables 5 and 6 ) (Fig. 4 ). Cancer cells in dose-dependent manner. Complex 4 exhibits cytotoxic effect on BT474 cancer cells but no antiproliferative effect against cell line HOP62. The antiproliferative effect of tested complexes is likely due to the lipophilicity of the complexes that alleviate the transport of metal complexes into the cell and posteriorly into the organelles where metal may possibly contribute to toxicity by inhibitory cellular respiration and metabolism of biomolecules [ 57 , 58 , 59 ]. The pure metals are inactive however the activity of metal cations varies on their bioavailability hence delivery methods/solubility and ionization of metal sources are significant parameters to deal metals in biological system [ 60 , 61 , 62 ] possibly this is the reason that bonding of metal cations (Cu(II)/Ni(II)/Co(II) to biologically compatible ligand (FGAA) enhances the bioavailability and ultimately the activity of metal cations. In contrast, coordination enhances the activity of the metal complexes against BT 474 and HOP 62 cell lines. The compound 1 (ligand) exhibited no cytotoxic effect on both the cell lines. The choice of the coordinated ligand (s) seems to be as important as the choice of metal(s) because besides being the integral part of biologically active complexes. These organic molecules (ligands) can exert a biological activity of their own. The photomicrograph of the cells treated with compounds 1, 2, 3, and 4 revealed the morphological features of apoptosis consist of membrane blebbing, nuclear condensation, cytoplasmic shrinkage, DNA fragmentation, cell wall destruction, and formation of apoptotic bodies (Figs. 5 and 6 ). Morphological images were grabbed using a phase-contrast microscope at × 20 magnifications with a digital camera at 48 h after treatment with the samples. Compound 1 showed negligible cytotoxicity as the cell growth and morphology did not get affected whereas it can be seen clearly that the compounds 2 , 3 , and 4 affected the normal morphology which rendered the cells to lose their viability. The picture revealed that the cells treated with compounds 2 , 3 , and 4 exhibited apoptotic cellular death as the population of cells reduced drastically within the 48 h of treatment. The photomicrograph depicts the treatment of HOP 62 and BT 474 cells treated with complexes 2 , 3 , and 4 showed a significant inhibitory effect on the cellular growth. In all two cell lines, the increase in the number of cells with abnormal morphology was accompanied by an increase in the number of irregular refractive clumps. The majority of the cell appear to be rounded and shrunken due to apoptosis and white spots in the images showing the apoptotic cell. The percentage cell viability in presence of ligand and metal complexes for BT 474 and HOP 62 cell lines are shown in Figs. 3 and 4 . The graphs of percentage control growth versus molar drug concentration showed the effective drug concentration on both cell lines and each point is the mean standard error obtained from three independent experiments. The results showed that complexes 2 , 3 , and 4 were the most potent and strongly inhibited the proliferations of both the two cell lines in a dose-dependent manner with TGI values 17.3, 30.3, and 46.4 μg/ml respectively. It was verified that the increased in concentration of complexes leads to higher cytotoxic activities. Nevertheless, results also proved that ADR showed superior cytotoxic activity against both cell lines.
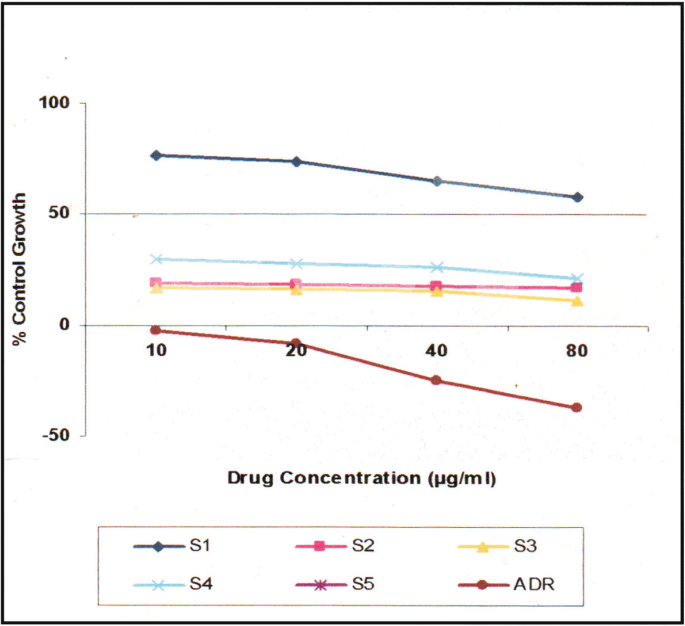
Growth curve: human lung cancer cell line HOP 62 compounds 1-4
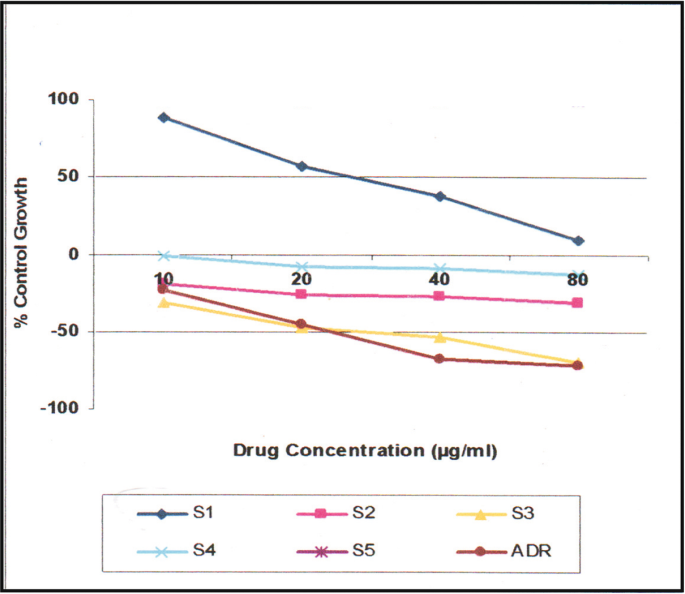
Growth curve: human breast cancer cell line BT 474 compounds 1 - 4
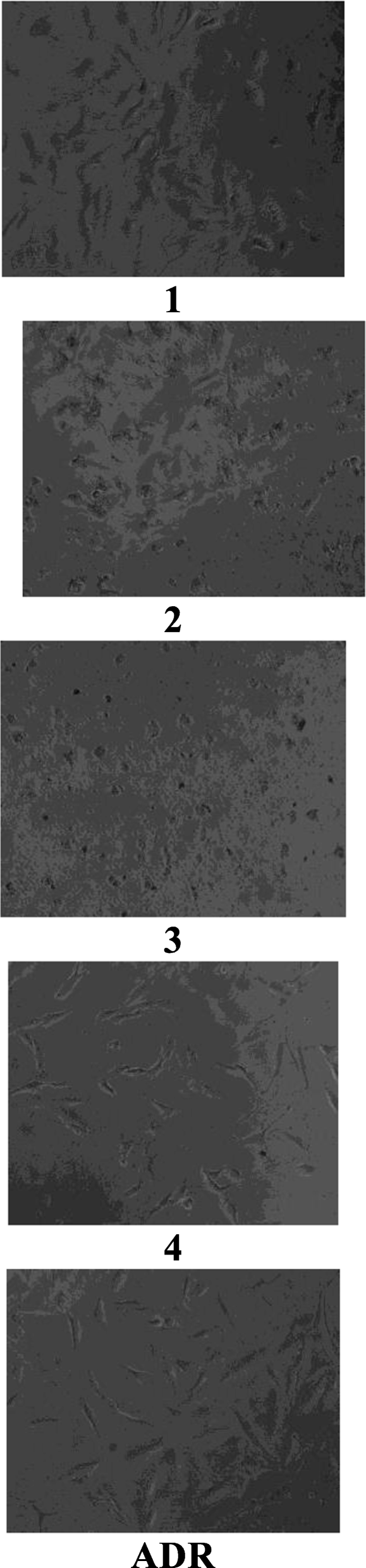
HOP 62 Cell images compounds 1 - 4
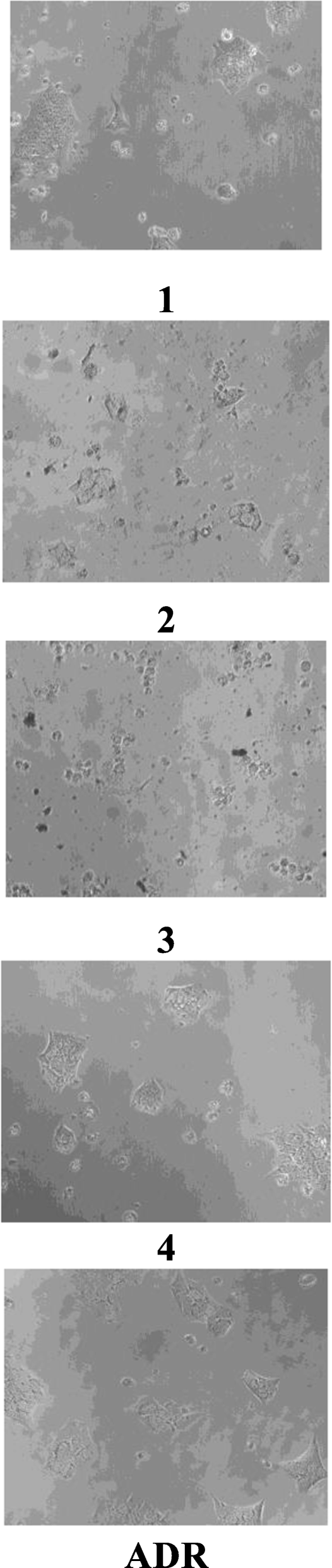
BT474 Cell images compounds 1-4
Fluorescence studies
Emission intensity of the three complexes increases on increasing the conc. of CT DNA. The enhancement of emission intensity is an indication of binding for the complexes to the hydrophobic pockets of DNA and complexes can be protected efficiently by the hydrophobic environment inside the DNA helix [ 63 , 64 ]. The high binding affinities of the metal complexes are probably attributed to the extension of the π system of the intercalated ligand due to the co-ordination of transition metal ions which also leads to a planar area greater than that of the free ligand and the coordinated ligand penetrating more deeply into and stacking more strongly with base pairs of DNA. The quenching plots illustrate that the quenching of ethidium bromide (EtBr) bound to DNA by the complexes are in good agreement with the linear Stern-Volmer equation which proves that the three complexes bind to DNA. The K b (slope/intercept values in graph of F 0 / F vs (conc.) values for complexes 2 , 3 , and 4 are 2.225 × 10 2 M –1 , 1.94 × 10 2 M –1 , and 1.55 × 10 2 M –1 ). Based on the K b values, the order of binding strength of metal complexes 2 > 3 > 4 (Figs. 7 , 8 , 9 ).
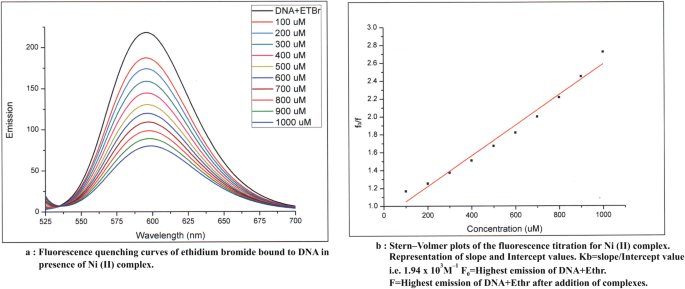
a Fluorescence quenching curves of ethidium bromide bound to DNA in presence of Ni (II) complex. b Stern-Volmer plots of the fluorescence titration for Ni (II) complex. Representation of slope and Intercept values. Kb = slope/Intercept value i.e. 1.94 × 10 2 M -1 F0 = Highest emission of DNA+Etbr, F = Highest emission of DNA+Etbr after addition of complexes
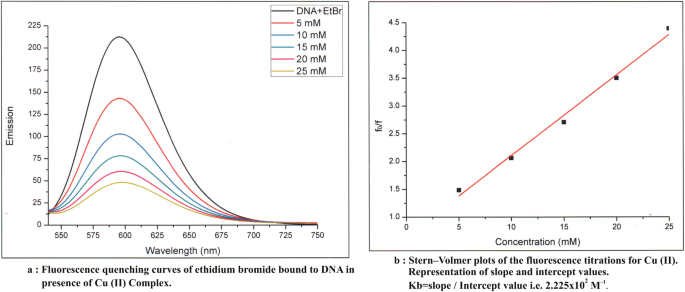
a Fluorescence quenching curves of ethidium bromide bound to DNA in presence of Cu (II) Complex. b Stern-Volmer plots of the fluorescence titrations for Cu (II) Complex. Representation of slope and intercept values. Kb = slope/Intercept value i.e. 2.225 × 10 2 M -1
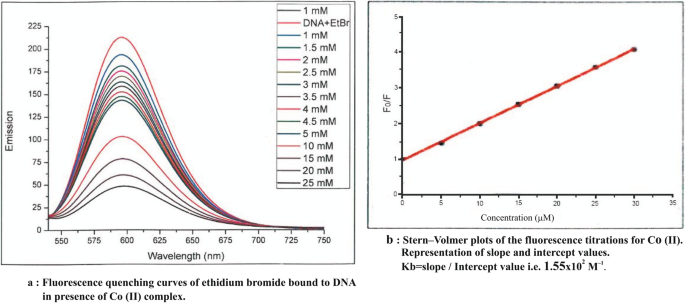
a Fluorescence quenching curves of ethidium bromide bound to DNA in presence of Co (II) complex. b Stern-Volmer plots of the fluorescence titrations for Co (II) Complex. Representation of slope and intercept values. Kb = slope/Intercept value i.e. 1.55 × 10 2 M -1
Conclusions
In the present study, novel metal complexes of Cu(II), Ni(II), and Co(II) were prepared and characterized by physico-chemical methods. Spectral studies demonstrate the ligand coordinating through azomethine nitrogen and carboxylate oxygen atoms and reveal octahedral geometry for Cu(II), Ni(II), and Co(II) complexes. The antibacterial and antifungal data given for the compound presented in this paper allowed us to state that the metal complexes generally have better activity than the ligands and less activity than standards. The metal complexes exerted growth inhibition on the human tumor cell lines showing promise as potential anticancer drugs deserving of further investigation. The Schiff base exhibits a strong fluorescence emission contrast to this partial fluorescence quenching phenomena is observed in its metal complexes.
Availability of data and materials
Available on request
Abbreviations
2-Furylglyoxal-anthranilic acid
Tera methyl silane
Dimethyl formamide
Dimethyl sulfoxide
Minimum inhibitory concentration
Sulforhodamine B
50% growth inhibition
Total growth inhibition
Cytotoxic killing of 50% of cells
Patole J, Dutta S, Padhye S, Sinn E (2001) Inorg Chim Acta 318:207
Article CAS Google Scholar
Maurer RI, Blower PJ, Dilworth JR, Reynolds CA, Zheng Y, Mullen GED (2002) J Med Chem 45:1420
Article CAS PubMed Google Scholar
Jouad EM, Thanh XD, Bouet G, Bonneaus, Khan MA (2002) Anti Cancer Res 22:1713
CAS Google Scholar
Cowly AR, Dilworth JR, Donnely PS, Labisbal E, Sousa A (2002) J Am Chem Soc 124:5270
Gray HB (2003) Proc Natl Acad Sci U S A 100:3563
Article CAS PubMed PubMed Central Google Scholar
Abrams MJ, Murrer BA (1993) Science 261:725
Rosenberg B, Vancamp L, Troska JE, Mansour VH (1969) Nature 222:385
Guo Z, Sadler P (2000) J Adv Inorg Chem 49:183–306
Cvek B, Milacic V, Taraba J, Dou QP (2008) J Med Chem 51:6256
Anderson RF, Denny WA, Ware DC, Wilson WR (1996) Br J Cancer 74:548
Article Google Scholar
Teicher B, Abrams M, Rosbe K, Herman T (1990) Cancer Res 50:6971
CAS PubMed Google Scholar
Ware DC, Palmer HR, Brothers PJ, Rickard CEF, Wilson WR, Denny WA (1997) J Inorg Bichem 68:215
Wilson WR, Moselen JW, Cliffe S, Denny WA, Ware DC (1994) Int J Radiat Oncol Biol Phys 29:323
Ware DC, Palmer BD, Wilson WR, Denny WA (1993) J Med Chem 36:1839
Vogel AI, Furniss BS (1989) A text book of practical inorg chem, 5th edn. Longman, London
Google Scholar
Kipnis F, Ornfelt J (1948) J Am Chem Soc 70:3948
Cai YH (2012) Asian J Chem 24(10):4468–4470
Elzahany E, Hegab K, Khalil S, Youssef N (2008) Aust J Basic Sci 2(2):210–220
Wang Q, Wang Y, Yang ZY (2008) J Chem Pharm Bull 56(7):1018–1021
Yong Feng Q, Lin D, Zhou J, Hu Y, Lin L, Li B, Qihua Z (2014) J Coord Chem 67(15):2615–2629
Vogel AI (1978) A text book of quantitative inorganic analysis Longman, London
Ferraari MB, Capacchis S, Reffo G, Tarasconi P, Albertini R, Pinelli S, Lunghi P (1999) Inorg Chim Acta 286:134–141
Pandaya SN, Sriram D (1999) Declercq. Eur J Pharm Sci 9:25–31
Bauer AW, Kirby WM, Sherris JC, Truck M (1966) Am J Clin Pathol 45:493–496
Avishai BD, Charles E, Davidson (2014) J Microbiol Methods 107:214–221
Hedges AJ (2002) Int J Food Microbiol 76:2002
Cullen JJ, Maclntyre HL (2016) J Appl Psychol 28(1):279–298
Fricker SP, Buckley RG (1996) Anticancer Res 16:3755–3760
Keepers YP, Pizao PE, Peters GJ, Arkeotte J, Winograd B, Pinedo HM (1991) Eur J Cancer 27:897–900
Papazisis KT, Geromichalos GD, Dimitriadis KA, Kortsaris AH (1997) J Immunol Methods 208:151–158
Griffon G, Merlin JL, Marchal C (1995) Anti-Cancer Drugs 6:115–123
Mosmann T (1983) J Immunol Methods 65(1-2):55–63
Jin M, Zhao W, ,Zhang Y, Kobayashi M, Duan H, Kong D (2011) Int J Mol Sci 12(11): 7352-7359.
Hui Y, Wei Z, Qing Y, Fuping H, Hedong B, Hong L (2017) Molecules 22(10):3–10
Xiao YJ, Diao QC, Liang YH, Zeng K (2017) Braz J Med Biol Res 50:1–5
Wang FY, Tang XM, Wang X, Huang KB, Feng HW, Chen ZF, Liu YN, Liang H (2018) Eu J Med Chem 155:639–650
Latif MA, Tofaz T, ,Zahan KD (2019) Russ J Chem 89:1197-1201.
Iskander MF, Eisyed L, Ismail KZ (1979) Transit Met Chem 4:225
Thankamony M, Mohanan K (2007) Indian J Chem A 46:249
Thomas M, Nair MKM, Radhakrishan RK (1995) Synth React Inorg Met-Org Chem 25:471
Nakamoto K (1997) Infrared and Raman spectra of inorganic and coordination compounds. Wiley, New York
Xiu RB, Mintz FL, You XZ, Wang RX, Yue Q, Meng QJ, Luy J, Derveer DV (1996) Polyhedron 15:4585
Lever ABP (1968) Inorganic electronic spectroscopy. Elsevier, New York
Sharda LN, Ganorkar M (1988) Indian J Chem A 27:617
Warad DV, Satish CD, Kulkarini VH, Bajgur CS (2000) Indian J Chem A 39:415
Skoog DA, Holler F, James C, Stanley R (2007) Principles of instrumental analysis, 6th edn. Thomson Brooks Cole, Belmont, pp 169–173
Reddy PM, HO YP, Shankar K, Rohini R, Ravinder V (2009) J Coord Chem 44:2621–2625
Prasad AV, Reddy PM, Shankar K, Rohini R (2009) Color Technol 125:284–287
Shankar K, Rohini R, Reddy PM, HO YP, Ravinder V (2009) J Coord Chem 62:3040–3049
Bhave NS, Kharat RB (1980) J Inorg Nucl Chem 45:977
Gunther H (1995) NMR spectroscopy basic principles, concepts, and applications in chemistry, 2nd edn. Wiley
Freeman RA (1997) Handbook of nuclear magnetic resonance, Longman, Essex, 2nd ed.UK
Irobi ON, Moo-Young M, Anderson WA (1996) Int J Pharm 34:87
Pelczar MJ, Chan ECS, Krieg NR (1998) Microbiology Blackwell Science, New York
Win Y, Yousif E, Majeed A, Ha S (2011) Asian J Chem 23(11):5009–5012
Stokes EJ, Ridgway GL (1980) Clinical Bacteriology Edward Arnold Publisher Maryland USA
Singh AP, Kaushik NK, Verma AK, Hundal G, Gupta R (2009) Eur J Med Chem 44:2009
Verma AK, Bansal S, Singh J, Tiwari RK, Kasi Sonkar V, Tandon V, Chandra R (2006) Bio Org Med Chem 14:6733
Takara K, Obata Y, Yoshikawa E, Kitada N, Sakaeda T, Ohnishi N, Xokoyama T (2006) Cancer Chemother Pharmacol 58:785
Jevtovic V, Pelosi G, Ianelli S, Kuvacevic R, Kaisarevic S (2010) Acta Chim Slov 57:363–369
Loa J, Chow P, Zhang K (2009) Cancer Chemother Pharmacol 63:1007
Jiang S, Yan G, Way J (2000) J Chem Soc Dalton Trans:1431
Lakowicz JR (1999) Principles of fluorescence spectroscopy, 2nd edn. Kluwer Academic Press Planum Publishers, New York
Book Google Scholar
Chem GJ, Huang XZ, Zheng ZZ, XU JG, Wang ZB (1990) Methods of fluorescence analysis, 2nd edn. Science Press, Beijing
Download references
Acknowledgements
The author express his gratitude to the STIC, Cochin University, CDRI, Lucknow , S.P Centre for Science and Technology Vallabh Vidhya Nagar, and ACTREC Mumbai for providing facilities of spectral and biological studies.
Not applicable
Author information
Authors and affiliations.
Chemical Research Laboratory, Faculty of Science, Department of Chemistry, DS College Aligarh, Dr. BR Ambedkar University, Agra, India
Vinay Kumar Srivastava
You can also search for this author in PubMed Google Scholar
Contributions
The author designed, performed experiments, analyzed data, and wrote the manuscript. The author read and approved the final manuscript.
Corresponding author
Correspondence to Vinay Kumar Srivastava .
Ethics declarations
Ethics approval and consent to participate, consent for publication, competing interests.
The author has no conflict of interest.
Additional information
Publisher’s note.
Springer Nature remains neutral with regard to jurisdictional claims in published maps and institutional affiliations.
Rights and permissions
Open Access This article is licensed under a Creative Commons Attribution 4.0 International License, which permits use, sharing, adaptation, distribution and reproduction in any medium or format, as long as you give appropriate credit to the original author(s) and the source, provide a link to the Creative Commons licence, and indicate if changes were made. The images or other third party material in this article are included in the article's Creative Commons licence, unless indicated otherwise in a credit line to the material. If material is not included in the article's Creative Commons licence and your intended use is not permitted by statutory regulation or exceeds the permitted use, you will need to obtain permission directly from the copyright holder. To view a copy of this licence, visit http://creativecommons.org/licenses/by/4.0/ .
Reprints and permissions
About this article
Cite this article.
Srivastava, V.K. Synthesis, characterization, and biological studies of some biometal complexes. Futur J Pharm Sci 7 , 51 (2021). https://doi.org/10.1186/s43094-021-00191-w
Download citation
Received : 26 August 2020
Accepted : 21 January 2021
Published : 24 February 2021
DOI : https://doi.org/10.1186/s43094-021-00191-w
Share this article
Anyone you share the following link with will be able to read this content:
Sorry, a shareable link is not currently available for this article.
Provided by the Springer Nature SharedIt content-sharing initiative
- Metal complexes
- Characterization
- Antimicrobial
- Anticancer activity
- DNA binding

An official website of the United States government
The .gov means it’s official. Federal government websites often end in .gov or .mil. Before sharing sensitive information, make sure you’re on a federal government site.
The site is secure. The https:// ensures that you are connecting to the official website and that any information you provide is encrypted and transmitted securely.
- Publications
- Account settings
Preview improvements coming to the PMC website in October 2024. Learn More or Try it out now .
- Advanced Search
- Journal List

Organic Synthesis via Transition Metal-Catalysis
In recent years, the development of transition-metal-catalyzed reactions has acquired an increasing importance. In fact, the use of transition metal complexes as catalysts may allow performing important organic transformations in one synthetic step by the assembly of simple units under sustainable conditions [ 1 ]. This Special Issue reports significant contributions in this field. In particular, seven papers have been published: two reviews and five original research articles.
The first review (by Garazi Urgoitia, Maria Teresa Herrero, Fátima Churruca, Nerea Conde, and Raul SanMartin) reports on the use of palladium pincer complexes as catalysts or pre-catalysts for the direct arylation of arenes with aryl halides or pseudo-halides [ 2 ]. This is a particularly important process, which allows aryl–aryl coupling (also intramolecularly) using an arene (or heteroarene) as one coupling partner, with C–H activation.
The second review (by Mieko Arisawa and Masahiko Yamaguchi) concerns the synthesis of a plethora of organosulfur compounds by rhodium-catalyzed S–S bond cleavage of disulfides or elemental sulfur and subsequent transfer of the ensuing organothio groups to organic substrates [ 3 ].
In the first original research article, Jérémy Ternel, Adrien Lopes, Mathieu Sauthier, Clothilde Buffe, Vincent Wiatz, Hervé Bricout, Sébastien Tilloy, and Eric Monflier describe the reductive hydroformylation (performed under 80 bar pressure of a 1:1 CO/H 2 mixture, in toluene at 80 °C) of isosorbide diallyl ether (readily available by allylation of bio-sourced isosorbide). The process is catalyzed by a rhodium/amine catalytic system, [typically, Rh(acac)(CO) 2 /Et 3 N], to give the corresponding high value-added bis-primary alcohols [ 4 ].
The second original research article (by Joanna Palion-Gazda, André Luz, Luis R. Raposo, Katarzyna Choroba, Jacek E. Nycz, Alina Bieńko, Agnieszka Lewińska, Karol Erfurt, Pedro V. Baptista, Barbara Machura, Alexandra R. Fernandes, Lidia S. Shul’pina, Nikolay S. Ikonnikov, and Georgiy B. Shul’pin) reports on the use of methyl-substituted 8-hydroxyquinolines (Hquin) for the preparation of five-coordinated oxovanadium(IV) complexes [VO(2,6-(Me) 2 -quin) 2 , VO(2,5-(Me) 2 -quin) 2 , and VO(2-Me-quin) 2 ]. These complexes were then used as catalysts for the efficient oxidation of hydrocarbons (to alcohols) and alcohols (to ketones), carried out with H 2 O 2 in acetonitrile at 50 °C in the presence of 2-pyrazinecarboxylic acid (PCA) as a cocatalyst [ 5 ].
The subsequent papers report on transition-metal-promoted cyclization processes leading to heterocyclic derivatives. Thus, new polycyclic heterocycles (1 H -benzo[4,5]imidazo[1,2- c ][1,3]oxazin-1-ones) were synthesized by Lucia Veltri, Roberta Amuso, Marzia Petrilli, Corrado Cuocci, Maria A. Chiacchio, Paola Vitale, and Bartolo Gabriele using a ZnCl 2 -promoted deprotective annulation approach starting from N -Boc-2-alkynylbenzimidazoles under mild conditions (CH 2 Cl 2 as the solvent at 40 °C for 3 h) [ 6 ]. N -benzoylindoles were obtained by Zhe Chang, Tong Ma, Yu Zhang, Zheng Dong, Heng Zhao, and Depeng Zhao by Pd(II)-catalyzed oxidative C–H functionalization and annulation of substituted N -(2-allylphenyl)benzamides, carried out with Pd(OAc) 2 as the catalyst in the presence of benzoquinone as the oxidant and dibutyl phosphate as the additive in DMSO at 60–70 °C [ 7 ]. An annulative C–H activation process was also developed by Bao Wang, Xu Han, Jian Li, Chunpu Li, and Hong Liu for the synthesis of fused isochromeno-1,2-benzothiazine derivatives, starting from S -phenylsulfoximides and 4-diazoisochroman-3-imine. The process, catalyzed by [Cp*RhCl 2 ] 2 in the presence of AgOPiv, takes place in trifluoroethanol at room temperature under air [ 8 ].
Conflicts of Interest
The author declares no conflict of interest.
Publisher’s Note: MDPI stays neutral with regard to jurisdictional claims in published maps and institutional affiliations.
- Publications
Transforming Energy Demand
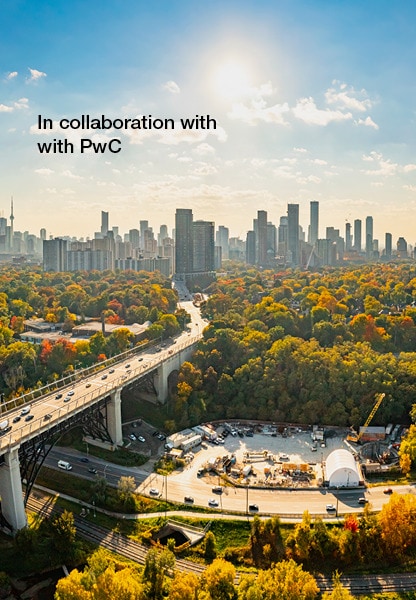
In this paper, the Transforming Energy Demand initiative outlines actions for businesses and countries to enhance energy management, efficiency and carbon intensity reduction. It highlights commercially beneficial levers, implementable with existing technologies, to impact the transition significantly.
Adopting measures for energy-efficient output and service delivery is essential for businesses and countries to sustain economic growth and achieve net-zero goals.
As the global population and energy demand rise, particularly in developing markets, implementing public policies and fostering value chain collaborations are key to managing energy consumption and reducing carbon intensity. This will help mitigate energy costs and supply issues and unlock commercial benefits, thereby accelerating the transition. At COP28, over 120 countries committed to doubling the pace of energy efficiency improvement, necessitating concrete, realistic plans.
World Economic Forum reports may be republished in accordance with the Creative Commons Attribution-NonCommercial-NoDerivatives 4.0 International Public License , and in accordance with our Terms of Use .
Further reading All related content
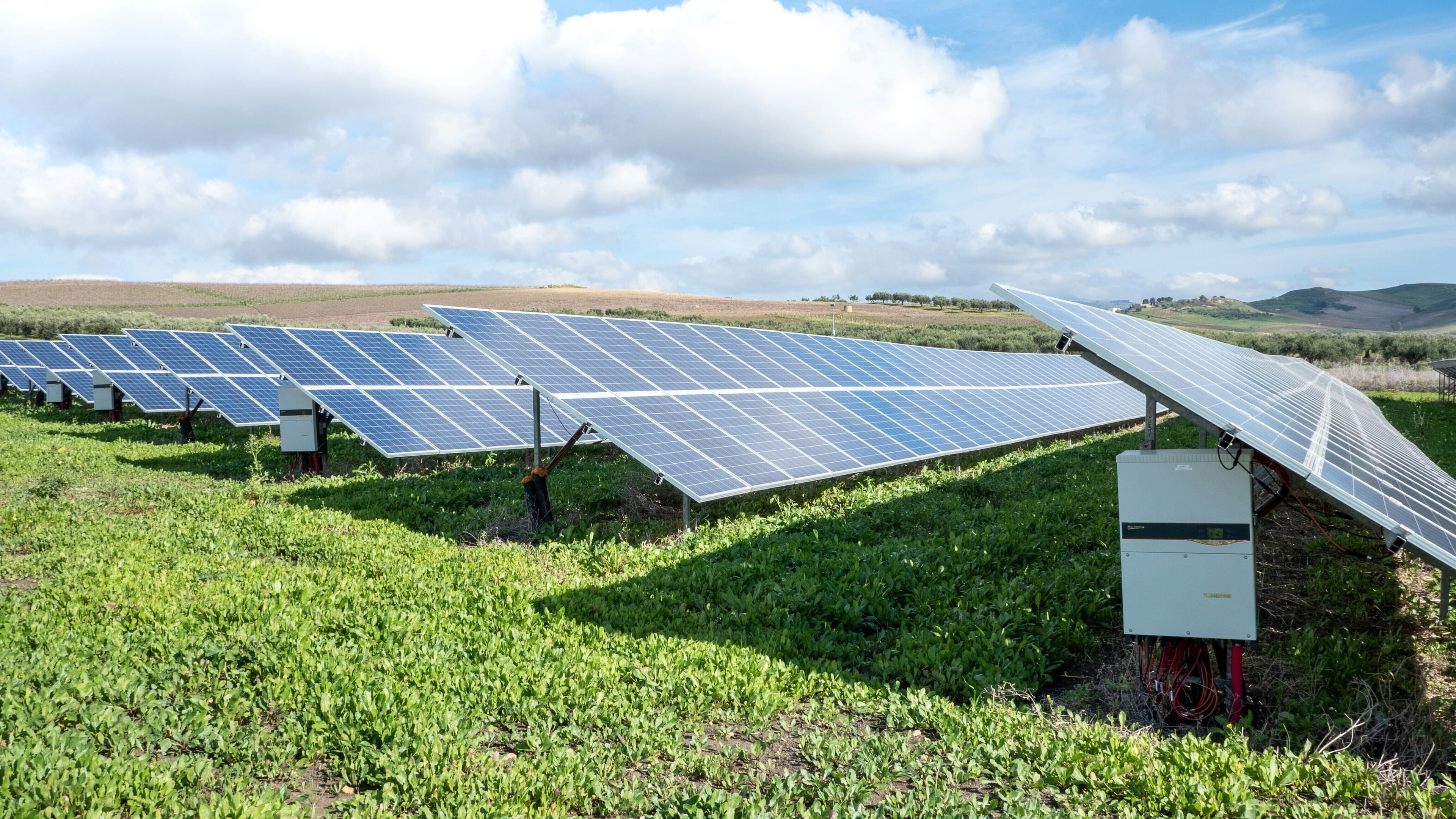
Cómo Davos 2024 fijó la agenda para acelerar una transición energética justa y rentable
La transición a la energía verde debe ser equitativa y justa, y el capital debe dirigirse a los más necesitados. Si se hace bien, podemos ahorrar billones y triplicar la energía limpia.
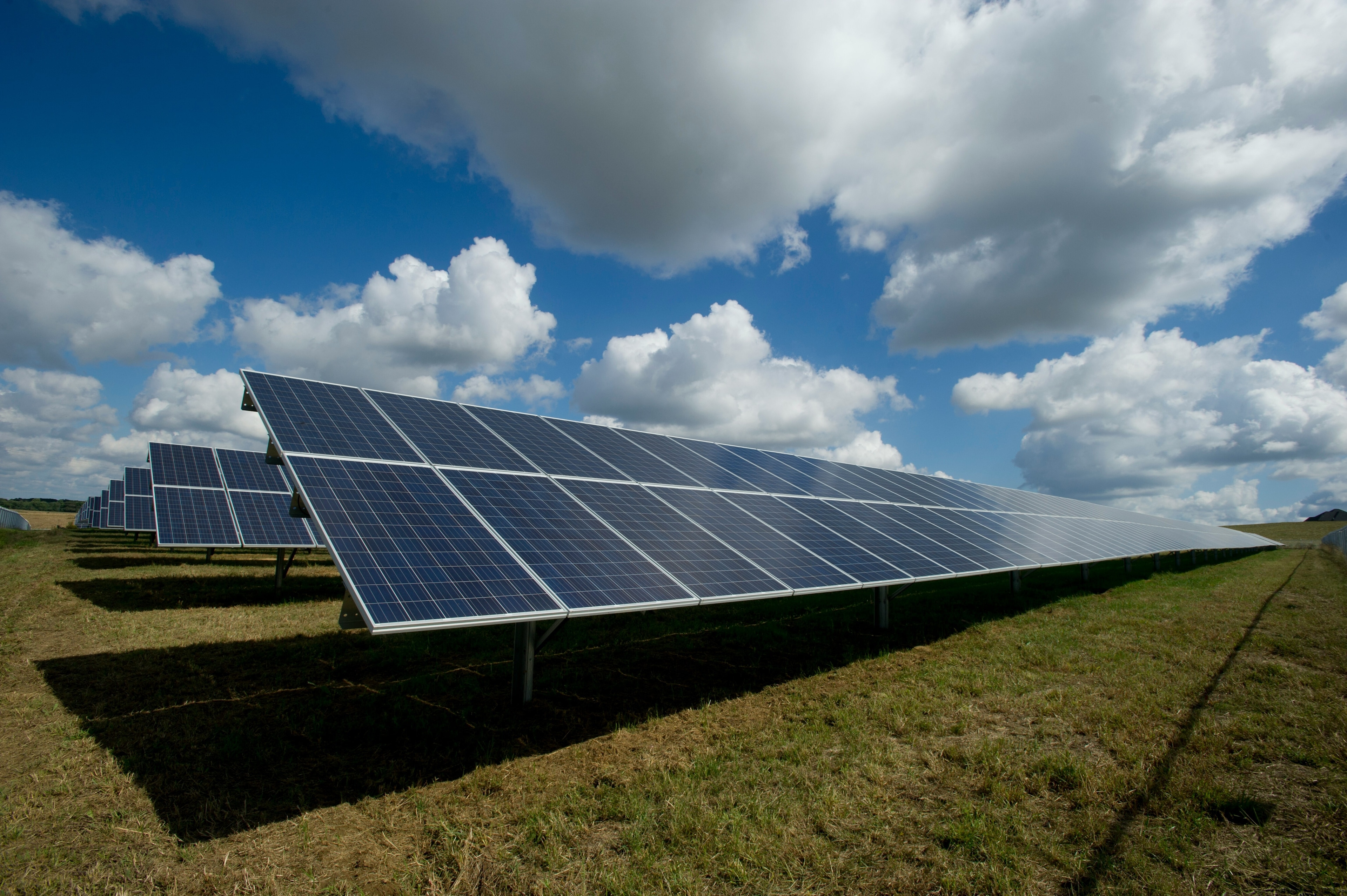
The world added 50% more renewable capacity last year than in 2022
How much is global renewable energy capacity increasing and what must happen to achieve the COP28 pledge to triple clean energy capacity by 2030?
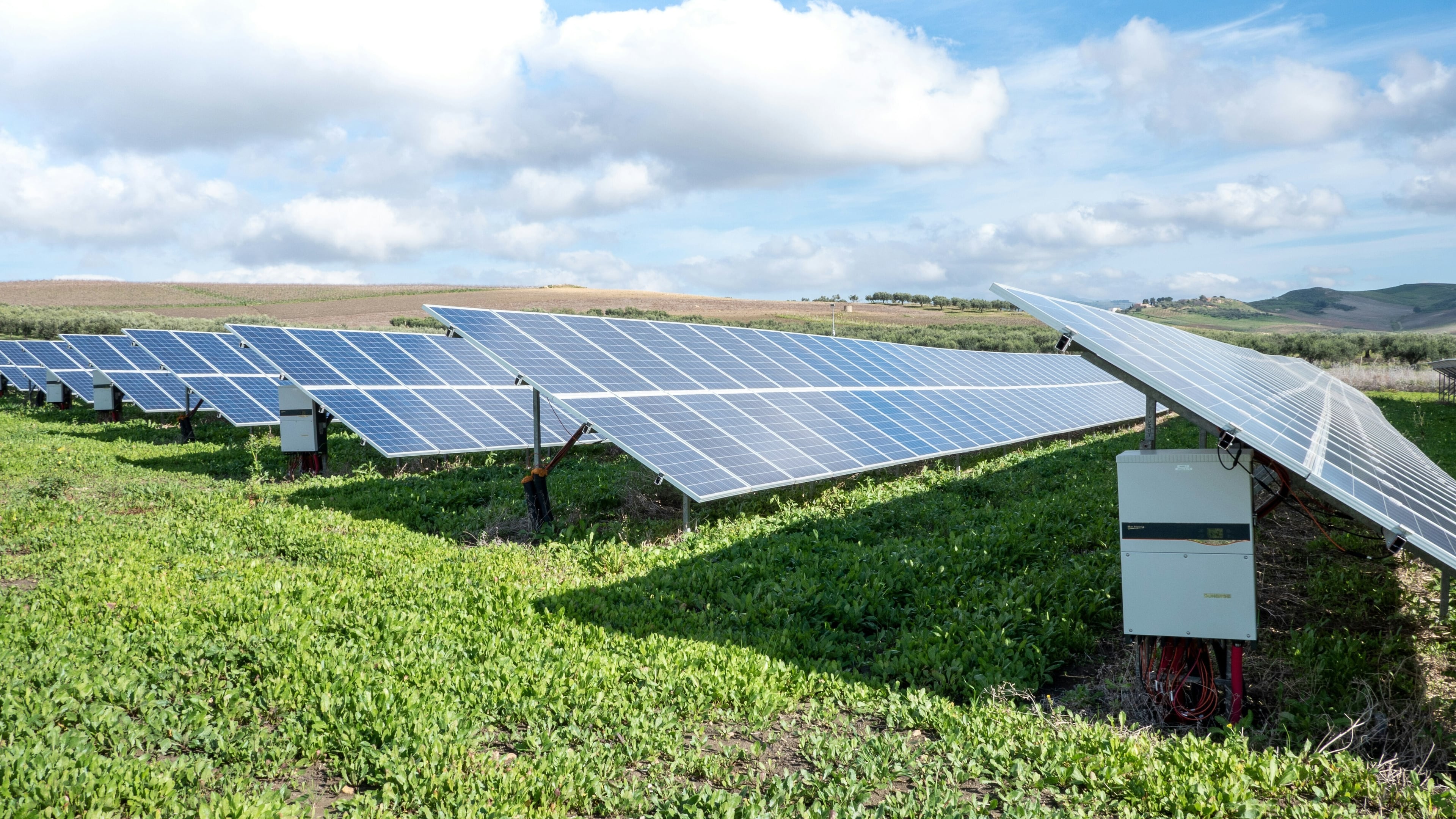
How Davos 2024 set the agenda for accelerating the energy transition in a fair and cost-effective way
The green energy transition must be equitable and fair, with capital directed to the most needed. If done right, we can save trillions and triple clean energy.
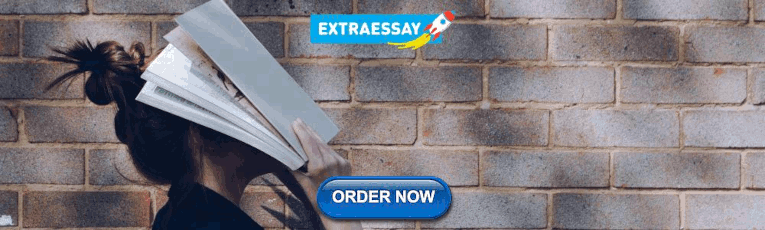
IMAGES
VIDEO
COMMENTS
Transition-metal complexes are attractive targets for the design of catalysts and functional materials. The behavior of the metal-organic bond, while very tunable for achieving target properties, is challenging to predict and necessitates searching a wide and complex space to identify needles in haystacks for target applications. This review will focus on the techniques that make high ...
Transition metal-based oxides gained much research interest in the field of energy storage and conversion technologies due to their low cost, earth abundance, and high corrosion resistance. Owing to the various oxidation states of transition metals they act as best OER electrocatalysts [15] and it is proved that the transition metals with ...
Transition metal oxides, in addition to elemental precursors, could be utilized in the synthesis process. ... 2D TMD-based biosensors with enhanced sensitivity, selectivity, quick response, and fewer power consumptions. Although research into 2D TMDs and TMD-based heterostructures is still in its early phases, newer methodologies and platforms ...
Introduction. Two-dimensional transition metal dichalcogenides (TMDCs) are an emerging class of materials with properties that make them highly attractive for fundamental studies of novel physical ...
ConspectusIn the wake of the discovery of the remarkable electronic and physical properties of graphene, a vibrant research area on two-dimensional (2D) layered materials has emerged during the past decade. Transition metal dichalcogenides (TMDs) represent an alternative group of 2D layered materials that differ from the semimetallic character of graphene. They exhibit diverse properties that ...
Apart from the above-mentioned articles, a few interesting research works canvassing different transition-metal-catalyzed reactions have also been presented in this issue. The group of Xuefeng Jiang has showcased a versatile approach of transition-metal-catalyzed introduction of hypervalent sulfur to access SO 2-containing
Research Article. Open Access. Ferromagnetic Order in 2D Layers of Transition Metal Dichlorides. Andrea Aguirre, Andrea Aguirre. Centro de Física de Materiales CSIC-UPV/EHU, 20018 Donostia-San Sebastián, Spain. CIC nanoGUNE-BRTA, 20018 Donostia-San Sebastián, Spain. Search for more papers by this author.
This Special Issue presents the latest research on transition-metal complexes and their applications, including characterization and property studies on structurally interesting metal complexes, applications of metal complexes in various transformation reactions and mechanistic studies. Dr. Yungen Liu. Guest Editor.
Compared with single-metal TMCs, constructing heterostructure is one of the effective ways to improve their catalytic activity [].Lin et al. [] introduced electro spinning and pyrolysis to design Fe 3 C-Mo 2 C/nitrogen-doped carbon (Fe 3 C-Mo 2 C/NC) heterogeneous nanofibers (HNFs) with tunable compositions, resulting in abundant Fe 3 C-Mo 2 C heterogeneous interface to achieve synergy in ...
Transition Metal Chemistry; past, present and future. This year of 2019 marks the thirtieth anniversary of my involvement with Transition Metal Chemistry (TMC). In 1989, I started work at the Nitrogen Fixation Laboratory, a government-funded research institute on the University of Sussex campus. I had completed my PhD at Sussex a few years ...
Thus, the NPs provide well-defined building units that allow independently addressing the influencing catalyst parameters of the transition metal NPs and the metal oxide support. 21 Thanks to improvements of colloidal NP synthesis, the development of transition metal NPs has extensively advanced over the past decades and allows for carefully tailoring various structural characteristics (such ...
Research of the nonlinear optical characteristics of transition metal dichalcogenides in the presence of photoactive particles, plasmonic nanocavities, waveguides, and metamaterials is still in its early stages. This investigation delves into the high-order harmonic generation (HHG) from laser induced plasma of MoS2 nanosheets in the presence of semiconductor photoactive medium such as CdSe ...
The majority of Schiff bases are attractive ligands since they construct complexation efficiently, with most transition metals owing to the tunable of the stereo-electronic structures [9], [10].Meanwhile, Schiff bases may also function as multifunctional ligands suitable for the coordination of a range of metallic ions with varying geometries and oxidization states.
A flat glass carbon electrode loaded with transition metal/carbon catalyst was used as the working electrode, and Li foil (400 μm thick with a diameter of 14 mm) was used as the counter electrode ...
The recent discovery of the spin supersolid candidate Na 2 BaCo (PO 4) 2 has stimulated a great deal of research on triangular-lattice transition-metal phosphates. Here we report a comprehensive study on the structural, magnetic, and magnetocaloric properties of polycrystalline Na 2 A T (PO 4) 2 (A = Ba,Sr; T = Co,Ni,Mn). X-ray and neutron diffraction measurements confirm that Na 2 Ba T (PO 4 ...
Transition metal-based materials such as oxides, sulfide, selenides, nitrides, phosphide, and carbides can store much more energy than carbon due to faradaic charge transfer process in the electrochemical process [23, 24].Due to their high theoretical specific capacitance (100-2200 Fg −1), abundant sources, and low cost, transition metal oxides have attracted great attention of the ...
The accurate determination of melting curves for transition metals is an intense topic within high pressure research, both because of the technical challenges included as well as the controversial data obtained from various experiments. This review presents the main static techniques that are used for melting studies, with a strong focus on the diamond anvil cell; it also explores the state of ...
The oxygen evolution reaction (OER) mechanisms using transition metal-based electrocatalysts are instrumental in providing novel insights into both natural and artificial energy conversion processes.
In this paper, the energy storage principles and research results of three main transition metal-based electrode materials (transition metal oxide (TMOs), Layered double hydroxides (LDHs) and transition metal sulfide (TMDs)) and the research progress of their composites are reviewed, and the future of transition metal-based electrode materials ...
In addition, advanced techniques for physical characterization of water oxidation intermediates are also introduced, for the purpose of providing information for establishing reliable methodologies in water oxidation research. The study of transition metal-based water oxidation electrocatalysts is instrumental in providing novel insights into ...
Research Progress of Transition Metal-Based Electrocatalytic Materials. 俊伊 陈. Published in Advances in Analytical… 2023. Chemistry, Environmental Science, Materials Science. Electrochemical water decomposition is a promising technology for sustainable. View via Publisher. pdf.hanspub.org. Save to Library. Create Alert.
Defect Engineering for Enhanced Electrocatalytic Oxygen Reaction on Transition Metal Oxides: The Role of Metal Defects. Jingxuan Zheng, Jingxuan Zheng. National Engineering Research Center of Industry Crystallization Technology, School of Chemical Engineering and Technology, Tianjin University, Tianjin, 300072 China ... Search for more papers ...
of novel 14- membered tetraazamacrocyc lic transition metal complexes: DNA cleavage and antimicrobial studies, Journal of the Chilean Chemical Society, 53 (3), 1568-1571.
In this manuscript, we design a class of transition metal subphthalocyanine molecules as electrocatalysts for NRR. The results indicate that the Nb, Mo, and Tc systems can effectively absorb and activate a N 2 molecule and reduce onset potentials (−0.50, −0.43, and −0.65 V, respectively). Among them, the Tc@C 24 H 12 N 6 molecule has exceptionally high selectivity for NRR with the ...
The chemistry of transition metal complexes has received considerable attention largely due to their catalytic and bioinorganic relevance. Such complexes are also important due to their potential biological activities such as antibacterial, antifungal, antimalarial, and antitumor [1,2,3,4].Medicinal inorganic chemistry is comparatively a new discipline which developed after the serendipitous ...
In fact, the use of transition metal complexes as catalysts may allow performing important organic transformations in one synthetic step by the assembly of simple units under sustainable conditions [ 1 ]. This Special Issue reports significant contributions in this field. In particular, seven papers have been published: two reviews and five ...
This white paper contains the findings of phase one of the Transforming Energy Demand initiative (2023/24). It demonstrates a compelling case for energy demand actions. Research and International Business Council (IBC) members' examples show the potential for around a 31% reduction in the amount of energy required for businesses to deliver products and services at attractive returns, requiring ...