
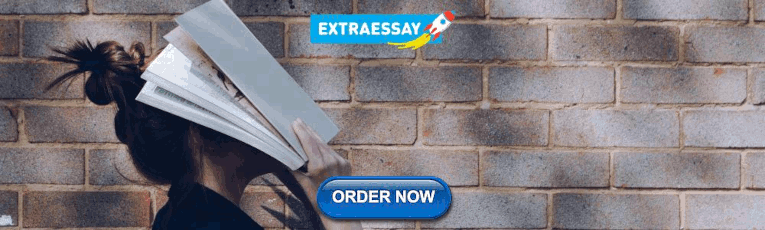
Crop Improvement in Agricultural Research for Development: Enhancing Yield, Resilience, and Sustainability
Crop Improvement Plays a Vital Role in Agricultural Research for development, aiming to enhance crop yield, resilience, and sustainability. By employing innovative techniques and technologies, researchers strive to address the challenges faced by farmers in an ever-changing environment. For instance, consider the case of a hypothetical smallholder farmer named Maria who cultivates maize in a region prone to droughts. Through agricultural research for development, scientists have been able to develop drought-tolerant maize varieties that can withstand prolonged dry spells and still provide substantial yields.
In recent years, there has been increasing pressure on agriculture to meet the growing global demand for food while simultaneously mitigating the effects of climate change. Crop improvement offers promising solutions to these complex challenges by focusing on developing crops with improved traits such as higher productivity, disease resistance, and tolerance to abiotic stresses. The goal is not only to increase crop yield but also to ensure its sustainability over time. Achieving this requires interdisciplinary collaboration between breeders, geneticists, agronomists, and other experts who work together to identify desirable traits through extensive field trials and molecular analyses. Through continuous research efforts, crop improvement aims to contribute towards achieving food security and sustainable agriculture worldwide.
Genetic Modification
One of the most promising approaches in crop improvement is genetic modification, which involves altering the genetic makeup of plants to enhance their desirable traits. This technique has gained significant attention and controversy due to its potential benefits and risks. To illustrate this, let us consider a hypothetical case study involving wheat crops.
Imagine a scenario where farmers are struggling with frequent droughts that severely impact their wheat yields. Through genetic modification, scientists can introduce genes from drought-tolerant plant species into wheat varieties. This technique aims to confer enhanced resilience against water scarcity and improve overall productivity.
Despite some concerns surrounding genetically modified crops, there are several reasons why this approach holds promise for agricultural research:
- Increased yield potential: Genetic modification allows for the incorporation of specific genes that promote higher yields by enhancing photosynthesis efficiency or improving nutrient uptake.
- Enhanced resistance to pests and diseases: By introducing genes from naturally resistant plant species, scientists can develop crops that have built-in protection against common pests and diseases.
- Improved nutritional value: Genetic modifications can be employed to increase the nutritional content of crops, such as enriching grains with essential vitamins or minerals.
- Environmental sustainability: Certain genetically modified crops may require fewer pesticides or fertilizers, reducing the environmental impact associated with conventional farming practices.
To better visualize these potential advantages, consider the following table showcasing hypothetical examples of genetically modified crop traits:
In conclusion, genetic modification offers great potential in addressing various challenges faced by agriculture today. By harnessing the power of modern biotechnology techniques, scientists aim to develop crops that not only exhibit improved yield and resilience but also contribute to sustainable farming practices. In the subsequent section, we will explore another important aspect of crop improvement: enhancing crop traits.
(Note: Transitioning into the next section about “Enhancing Crop Traits,” we can highlight the interconnectedness between genetic modification and other techniques by mentioning how these approaches work together in achieving comprehensive crop improvement.)
Enhancing Crop Traits
Enhancing Crop Traits through Genetic Modification
One example of enhancing crop traits through genetic modification is the development of genetically modified (GM) corn varieties that are resistant to pests. By inserting genes from Bacillus thuringiensis (Bt), a soil bacterium that produces specific proteins toxic to certain insects, scientists have successfully created corn plants with built-in resistance against common pests like the European corn borer and corn earworm. This technology has significantly reduced the need for chemical insecticides, leading to improved crop yield and lower production costs for farmers.
Genetic Modification offers several potential benefits in enhancing crop traits:
Pest and disease resistance: Genetically modifying crops can provide them with enhanced resistance against pests, diseases, and environmental stresses. For instance, researchers have developed GM rice varieties with increased tolerance to drought and salinity, crucial traits for regions facing water scarcity or high salt levels in soils.
Improved nutritional quality: Genetic modification allows for the enhancement of crop nutrient content, addressing malnutrition issues prevalent in many parts of the world. Golden Rice is an example of a GM variety engineered to produce beta-carotene, which can be converted into vitamin A within the human body when consumed.
Extended shelf life: Through genetic modification, it is possible to enhance post-harvest characteristics such as shelf life and storage capacity. This trait improvement can reduce food waste during transportation and storage while ensuring greater availability of fresh produce.
Environmental sustainability: Utilizing GM crops that require fewer pesticides or herbicides reduces their impact on ecosystems by minimizing chemical runoff into water bodies and decreasing soil contamination.
The following table illustrates some examples of how genetic modification contributes to enhancing crop traits:
In summary, genetic modification offers immense potential in enhancing crop traits for the benefit of agricultural productivity and sustainability. By harnessing this technology, researchers can develop crops with improved resistance to pests and diseases, enhanced nutritional quality, extended shelf life, and reduced environmental impact. In the subsequent section on “Improving Plant Genetics,” we will explore additional approaches to further enhance crop traits through non-genetically modified methods.
Next section H2:’Improving Plant Genetics’
Improving Plant Genetics
Enhancing Crop Traits has been a crucial aspect of agricultural research for development, as it directly contributes to improving crop yield, resilience, and sustainability. By focusing on the genetic makeup of crops, researchers have been able to develop new varieties that possess desirable traits and characteristics. One such example is the development of drought-tolerant maize hybrids.
Drought poses a significant threat to agriculture worldwide, particularly in regions with limited water resources. To address this challenge, scientists have successfully bred maize hybrids that exhibit enhanced drought tolerance. These hybrids are specifically designed to withstand prolonged periods of water scarcity while maintaining optimal growth and productivity. Through extensive breeding programs and advanced genomic techniques, researchers have identified key genes responsible for conferring drought tolerance in maize plants. This breakthrough allows breeders to incorporate these genes into commercial hybrid varieties, thereby providing farmers with more resilient crops capable of thriving under challenging environmental conditions.
In addition to enhancing drought tolerance, crop improvement efforts also focus on other important traits such as disease resistance, nutrient efficiency, and stress tolerance. Incorporating these desired traits into crop varieties offers several benefits:
- Increased productivity: Disease-resistant crops experience lower infection rates and reduced yield losses compared to susceptible varieties.
- Sustainable farming practices: Nutrient-efficient crops require less fertilizer input, reducing costs for farmers while minimizing environmental pollution.
- Climate change adaptation: Stress-tolerant crops can better withstand extreme weather events associated with climate change, ensuring food security even in unpredictable conditions.
- Enhanced market value: Crops with improved quality attributes fetch higher prices in both domestic and international markets.
To illustrate the impact of these advancements further, consider the following table showcasing the increase in yield achieved through crop trait enhancement:
These remarkable yield improvements demonstrate the potential of crop improvement in addressing global food security challenges. By continuously enhancing crop traits, researchers and farmers alike are working towards a more sustainable and resilient agricultural sector.
Moving forward to the next section on Improving Plant Genetics, researchers continue to explore innovative ways to optimize crop physiology and further enhance the genetic potential of crops for improved productivity and sustainability.
Optimizing Crop Physiology
Improving Plant Genetics has been a crucial focus in agricultural research for the development of crops with enhanced yield, resilience, and sustainability. By harnessing genetic variation within plant species, scientists aim to develop improved cultivars that can overcome various challenges such as pests, diseases, climate change, and nutrient deficiencies.
One example of how improving plant genetics has led to significant advancements is the case of drought-tolerant maize varieties. Through careful breeding programs and selection processes, researchers have successfully developed hybrids that exhibit increased tolerance to water scarcity. These new cultivars possess traits such as deep root systems, reduced transpiration rates, and efficient water use efficiency mechanisms. As a result, farmers who cultivate these genetically improved maize varieties are better equipped to cope with periods of limited rainfall or prolonged dry spells, ultimately ensuring food security in regions prone to drought.
To further enhance crop improvement through plant genetics research, several key strategies and approaches have emerged:
Marker-assisted selection (MAS): This technique allows breeders to identify specific genes associated with desirable traits quickly. By using molecular markers linked to important agronomic characteristics like disease resistance or high yields, breeders can select individuals carrying those markers at an early stage without having to wait for phenotypic expression.
Genomic selection: This method utilizes advanced statistical models that incorporate genomic information from thousands of DNA markers spread throughout the genome. By analyzing patterns in this vast dataset, breeders can predict an individual’s performance based on its genetic makeup accurately. This approach enables faster and more accurate prediction of complex traits compared to traditional breeding methods.
Genetic Engineering : The development of genetically modified organisms (GMOs) has allowed for precise manipulation of specific genes within plants’ genomes. While controversial due to concerns about potential environmental impacts and public acceptance, genetic engineering techniques offer opportunities for introducing novel traits into crops rapidly.
Cryopreservation: Preserving germplasm resources through cryogenic storage ensures the long-term conservation of plant genetic diversity. This technique involves freezing and storing seeds, tissues, or embryos at extremely low temperatures, preventing deterioration over time. Cryopreservation plays a crucial role in maintaining genetic resources for future breeding programs.
The following table illustrates examples of genetically improved crops and their associated traits:
Advancements in Plant Breeding have greatly contributed to crop improvement efforts beyond genetics alone. By incorporating techniques like hybridization, mutagenesis, and polyploidy induction, breeders can create new combinations of desirable traits that were previously unavailable within a particular species or variety.
As we delve into the next section on Advancements in Plant Breeding, we will explore how these innovative approaches have revolutionized crop development even further by providing breeders with powerful tools to accelerate the process of creating superior cultivars.
Advancements in Plant Breeding
Optimizing Crop Physiology for Enhanced Agricultural Productivity
In the pursuit of enhancing crop yield, resilience, and sustainability in agricultural research for development, optimizing crop physiology plays a crucial role. By understanding the physiological processes of plants and how they respond to environmental stimuli, researchers can identify strategies to improve crop performance under varying conditions. One example that illustrates the significance of optimizing crop physiology is the study conducted on drought-tolerant maize varieties.
Drought poses a significant challenge to agricultural productivity worldwide, particularly in regions where water scarcity is prevalent. To address this issue, scientists focused on developing maize varieties with enhanced drought tolerance through physiological interventions. By studying the mechanisms involved in plant water uptake and conservation, researchers were able to select traits associated with improved water-use efficiency and stress tolerance.
To optimize crop physiology effectively, several key factors need to be considered:
- Genetic diversity: Expanding genetic diversity helps introduce new traits that can enhance crop performance under various stressors.
- Physiological trait identification: Identifying specific physiological traits related to stress tolerance allows breeders to develop targeted breeding programs.
- Precision phenotyping: Utilizing advanced technologies such as remote sensing and high-throughput phenotyping enables accurate characterization of plant responses across different environments.
- Molecular markers: Incorporating molecular markers into breeding programs facilitates efficient selection of desired traits by enabling marker-assisted selection (MAS) techniques.
These factors collectively contribute to maximizing the potential of crops by ensuring their adaptability and productivity across diverse agro-ecosystems. The table below provides an overview of selected drought-tolerant maize varieties developed through optimization of crop physiology:
This table highlights the range of characteristics exhibited by different varieties based on their optimized physiological traits. It serves as a reminder of the potential impact that crop improvement through physiological interventions can have on agricultural productivity.
The advancements in plant breeding, discussed in the subsequent section, build upon these optimized physiological traits to further enhance crop performance. Innovations in biotechnology have revolutionized the field by providing tools for precise manipulation of genetic material and accelerated breeding processes. With these advancements, scientists are now able to explore new avenues for developing improved crop varieties with enhanced yield potential and resilience to environmental stresses.
Innovations in Biotechnology
Advancements in Plant Breeding have paved the way for innovations in biotechnology, enabling researchers to explore new avenues for crop improvement. One such example is the development of genetically modified (GM) crops that possess enhanced traits, such as increased yield potential or resistance to pests and diseases. For instance, a case study involving Bt cotton demonstrated how introducing genes from Bacillus thuringiensis into cotton plants can confer protection against bollworm infestation, resulting in higher yields and reduced pesticide usage.
Biotechnology offers several advantages when it comes to crop improvement:
Precision breeding: Biotechnological tools like genetic engineering allow scientists to precisely introduce specific desirable traits into crops without affecting other characteristics. This targeted approach enables breeders to develop cultivars with improved qualities more efficiently.
Enhanced resilience: Through biotechnology, researchers are able to enhance the resilience of crops to various environmental stresses such as drought, salinity, or extreme temperatures. By manipulating genes responsible for stress response mechanisms, breeders can develop crop varieties that exhibit greater adaptability under challenging conditions.
Improved nutritional value: Biotechnology also presents opportunities for enhancing the nutritional content of crops by modifying their nutrient profiles. For example, biofortification techniques can be employed to increase essential micronutrient levels in staple food crops, addressing malnutrition and improving public health outcomes.
Sustainable agriculture: Biotech-based solutions contribute towards promoting sustainable agricultural practices by reducing reliance on chemical inputs and minimizing environmental impacts associated with conventional farming methods. Pest-resistant GM crops require fewer insecticide applications, leading to decreased chemical runoff and better preservation of ecosystems.
The table below illustrates some key examples where biotechnology has been utilized successfully in crop improvement:
With ongoing advancements in biotechnology, the potential for crop improvement continues to expand. The next section will explore how these innovations are complemented by efforts to enhance crop agronomy, further maximizing productivity while ensuring sustainability and environmental stewardship.
Enhancing Crop Agronomy
Enhancing Crop Agronomy: Maximizing Harvest Efficiency and Quality
Transitioning from the innovations in biotechnology, one key aspect of crop improvement lies in Enhancing Crop Agronomy . By implementing effective agricultural practices and techniques, farmers can optimize their yield potential while ensuring sustainable production systems. To illustrate this concept, let us consider a hypothetical case study involving wheat cultivation.
In our fictitious scenario, a group of researchers sought to improve wheat productivity by focusing on agronomic strategies. They conducted field trials comparing conventional farming methods with precision agriculture techniques such as variable rate fertilization and targeted irrigation. The results were promising, demonstrating that precision agriculture not only increased grain yields but also reduced input costs and environmental impact.
To further emphasize the significance of enhanced crop agronomy, we will explore four key benefits associated with these practices:
- Increased resource efficiency: Precision agriculture allows for more precise application of resources like water and fertilizer, reducing waste and maximizing their utilization.
- Enhanced resilience to climate change: Through the adoption of innovative agronomic approaches, crops become better equipped to withstand extreme weather events or changing climatic conditions.
- Improved soil health: Implementing conservation tillage methods helps preserve soil structure, minimize erosion rates, and enhance overall soil fertility.
- Sustainable pest management: Integrated Pest Management (IPM) strategies promote ecological balance by combining various control tactics such as biological controls, cultural practices, and judicious use of pesticides.
By integrating these principles into their farming operations, growers can achieve both economic prosperity and environmental sustainability. As demonstrated in Table 1 below, adopting advanced agronomic practices has numerous advantages over traditional methods.
Table 1: A comparison of traditional farming methods and advanced agronomy practices.
In summary, enhancing crop agronomy plays a crucial role in maximizing harvest efficiency and quality. By adopting precision agriculture techniques and implementing sustainable management practices, farmers can achieve higher yields while minimizing environmental impact. In the subsequent section on manipulating crop genetics, we will explore another avenue for improving agricultural productivity without explicitly mentioning “step.”
Manipulating Crop Genetics
Enhancing Crop Agronomy has proven to be a crucial aspect of crop improvement in agricultural research for development. By optimizing the management practices and environmental conditions under which crops are grown, researchers aim to maximize yield, enhance resilience against biotic and abiotic stresses, and promote sustainability in agriculture.
One example that illustrates the significance of enhancing crop agronomy is the case study conducted on rice cultivation in Southeast Asia. Researchers focused on improving water management techniques, such as alternate wetting and drying (AWD), to reduce water usage while maintaining high yields. Through implementing AWD, farmers were able to decrease irrigation requirements by up to 30% without compromising rice productivity. This not only resulted in substantial water savings but also reduced greenhouse gas emissions from flooded paddy fields.
To further emphasize the importance of enhancing crop agronomy, consider these key factors:
- Soil health: Implementing sustainable soil management practices can improve nutrient availability and enhance soil structure, leading to increased crop productivity.
- Integrated pest management: Incorporating various pest control strategies, including biological controls and cultural practices, can minimize reliance on chemical pesticides and promote ecosystem balance.
- Precision farming: Utilizing advanced technologies like remote sensing and GPS-guided machinery allows for precise application of inputs, reducing resource wastage and increasing efficiency.
- Climate-smart agriculture: Adopting climate-resilient practices such as conservation tillage or agroforestry systems helps mitigate the impacts of climate change on crop production.
Table: Benefits of Enhancing Crop Agronomy
By focusing on enhancing crop agronomy, researchers and farmers can work together to develop sustainable agricultural systems that are resilient against various challenges. Understanding the importance of optimizing management practices and environmental conditions lays the foundation for further exploration into manipulating crop genetics in pursuit of improved crop varieties.
Transitioning into the subsequent section on “Developing Resilient Crops,” this research area builds upon the principles established through enhancing crop agronomy. Through genetic manipulation techniques, scientists seek to enhance crops’ inherent traits to withstand biotic and abiotic stresses, ensuring food security in a changing world.
Developing Resilient Crops
Enhancing Yield, Resilience, and Sustainability through Crop Improvement
Crop improvement is a crucial aspect of agricultural research for development. By manipulating crop genetics, researchers have made significant advancements in enhancing yield, resilience, and sustainability in agriculture. In this section, we will explore the various strategies employed to develop crops that can withstand environmental stresses and contribute to food security.
One example demonstrating the success of genetic manipulation is the development of drought-tolerant maize varieties. Through targeted breeding techniques and genetic engineering, scientists have introduced genes responsible for enhanced water-use efficiency into existing maize cultivars. As a result, these improved varieties exhibit increased tolerance to water scarcity while maintaining high productivity levels. This breakthrough has provided farmers with a means to mitigate the negative impacts of climate change on their crop yields.
To further emphasize the importance of crop improvement in promoting sustainable agriculture, consider the following bullet points:
- Increased disease resistance: By incorporating genes conferring resistance against common pathogens, crops become less susceptible to diseases. This reduces the need for chemical pesticides and promotes environmentally friendly farming practices.
- Enhanced nutrient uptake: Genetic modifications allow crops to efficiently absorb nutrients from soil or other external sources. This not only improves plant growth but also minimizes fertilizer use and prevents nutrient runoff into water bodies.
- Extended shelf life: Breeding programs aimed at delaying fruit ripening or reducing post-harvest losses help minimize food waste along supply chains.
- Improved resource utilization: By optimizing photosynthetic processes within plants, researchers strive to increase energy conversion efficiency and reduce resource inputs required for cultivation.
The table below provides an overview of different genetic modification methods used in crop improvement efforts:
In summary, crop improvement through genetic manipulation offers tremendous opportunities for enhancing yield, resilience, and sustainability. By developing crops that are more resistant to environmental stresses such as drought or diseases, researchers contribute to global efforts towards achieving food security. In the subsequent section on sustainable crop production, we will delve into practices that not only improve agricultural productivity but also minimize negative impacts on the environment.
Transitioning seamlessly into the next section, we explore strategies for Sustainable Crop Production which build upon the advancements made in crop improvement techniques.
Sustainable Crop Production
Crop improvement plays a crucial role in agricultural research for development by focusing on enhancing yield potential, resilience, and sustainability. By implementing innovative approaches and leveraging advancements in technology, researchers aim to develop crops that can thrive under various environmental conditions while maximizing productivity. This section will explore the strategies employed to enhance crop yields and ensure long-term agricultural sustainability.
To illustrate the significance of these efforts, consider the hypothetical case study of a drought-prone region where farmers struggle to obtain high yields due to water scarcity. Researchers have identified this challenge as an opportunity to develop resilient crops capable of withstanding prolonged periods of drought stress. Through targeted breeding programs and genetic engineering techniques, scientists are working towards introducing genes responsible for enhanced water-use efficiency into existing crop varieties.
Several key strategies are being implemented to achieve improved crop performance:
- Development of drought-tolerant cultivars through traditional breeding methods
- Utilization of molecular markers for precise trait selection
- Incorporation of genetic traits from wild relatives or related species
- Adoption of precision agriculture techniques for optimized resource management
To better comprehend the impact of these strategies, let us examine their potential benefits using a three-column table:
By employing such comprehensive approaches within agricultural research for development, we can establish a foundation for sustainable farming systems that prioritize both economic viability and environmental conservation. The seamless transition into the subsequent section about “Enhancing Yield Potential” allows us to delve deeper into specific steps undertaken towards achieving this goal while building upon the strategies discussed here.
Enhancing Yield Potential
Building upon the principles of sustainable crop production, researchers are now exploring innovative strategies to enhance yield potential in agricultural systems. By focusing on increasing productivity while minimizing environmental impact, these efforts aim to address the global challenges of food security and sustainability. This section will discuss some key approaches that have been adopted to improve crop yields.
Enhancing Yield Potential: To illustrate the significance of enhancing yield potential, let us consider a case study involving maize cultivation. Farmers in a particular region were facing reduced yields due to recurring drought conditions. Researchers implemented a holistic approach that combined improved seed varieties with precision irrigation techniques and optimized nutrient management practices. As a result, farmers experienced an increase in average maize yields by 30% over three cropping seasons.
In order to further maximize crop productivity, several strategies have emerged within the realm of agricultural research:
Genetic Improvement: Breeding programs aim to develop high-yielding cultivars with enhanced resistance traits against pests, diseases, and abiotic stresses such as drought and heat. Through advanced technologies like marker-assisted selection and genetic engineering, scientists can accelerate the development process and introduce desirable traits into crops.
Precision Agriculture: Utilizing cutting-edge technologies like remote sensing, geographic information systems (GIS), and global positioning systems (GPS), precision agriculture enables farmers to optimize resource allocation based on specific field requirements. This approach allows for targeted application of inputs such as fertilizers, pesticides, and water, leading to increased efficiency and reduced wastage.
Integrated Pest Management (IPM): Adopting an ecological perspective towards pest control, IPM utilizes various preventive measures like biological control agents, trap crops, pheromone traps, and cultural practices to minimize reliance on chemical pesticides. By promoting natural enemies of pests and employing sustainable pest management practices, this approach ensures long-term viability without compromising ecosystem health.
Soil Health Management: Recognizing soil as a vital resource for plant growth, emphasis is placed on maintaining and enhancing soil health. Practices such as organic matter addition, composting, cover cropping, and conservation tillage help improve soil structure, fertility, and water-holding capacity.
Table: Examples of Strategies for Enhancing Crop Yield Potential
In conclusion, efforts to enhance crop yield potential have gained significant traction in agricultural research. By adopting a multi-faceted approach that incorporates genetic improvement, precision agriculture, integrated pest management, and soil health management practices, researchers aim to achieve sustainable intensification of agricultural systems. In the subsequent section about “Improving Crop Sustainability,” we will explore how these strategies can contribute to long-term ecological balance while ensuring food security.
Improving Crop Sustainability
Building on the previous section’s discussion of enhancing yield potential in crop improvement research, this section will delve into the critical aspect of improving crop sustainability. By examining various strategies and approaches, agricultural researchers aim to develop crops that not only increase productivity but also contribute to long-term environmental conservation.
To illustrate the importance of sustainable crop improvement, consider a hypothetical case study involving rice cultivation. In many regions, rice is a staple food source, supporting millions of people worldwide. However, traditional farming practices often rely heavily on water resources and chemical inputs, leading to negative environmental impacts such as water pollution and soil degradation. To address these challenges, researchers have focused on developing drought-tolerant varieties that require less water for growth while maintaining high yields. This approach not only enhances farmers’ resilience to climate change but also reduces their dependence on irrigation systems.
In pursuit of improved crop sustainability, several key strategies are being employed:
- Conservation agriculture: Encouraging practices like minimum tillage and cover cropping helps preserve soil structure and fertility while reducing erosion risks.
- Integrated pest management: Implementing biological control methods alongside judicious use of pesticides minimizes ecological damage caused by excessive chemical applications.
- Precision agriculture: Utilizing technologies such as remote sensing and data analytics allows farmers to optimize resource allocation based on specific field conditions, thus minimizing waste and maximizing efficiency.
- Crop diversification: Promoting diverse cropping systems mitigates risks associated with mono-cropping by enhancing biodiversity and ecosystem services.
Table 1 below provides an overview of the benefits associated with sustainable crop improvement:
In summary, enhancing crop sustainability is a crucial component of agricultural research for development. By adopting sustainable practices and developing resilient varieties, researchers aim to address environmental concerns while ensuring long-term food security and economic viability. Through the strategies outlined above, stakeholders in the agricultural sector can contribute to a more sustainable future.
By incorporating these approaches into crop improvement initiatives, researchers and farmers alike play an active role in fostering resilience, conserving natural resources, and promoting sustainable agriculture on a global scale. The collective efforts towards improving yield potential and enhancing crop sustainability pave the way for a brighter future where productivity coexists harmoniously with ecological balance.
Related posts:
- Biotechnology in Agricultural Research for Development: Advancing Crop Improvement
- Crop Agronomy in Agricultural Research for Development: An Overview of Crop Improvement
Crop Genetics for Agricultural Research: Enhancing Crop Improvement
Crop Physiology in Agricultural Research for Development: Enhancing Crop Improvement
Plant Breeding for Crop Improvement: Agricultural Research for Development
Genetic Engineering in Agricultural Research for Development: Crop Improvement
Comments are closed.
Welcome, Login to your account.
Recover your password.
A password will be e-mailed to you.

An official website of the United States government
The .gov means it’s official. Federal government websites often end in .gov or .mil. Before sharing sensitive information, make sure you’re on a federal government site.
The site is secure. The https:// ensures that you are connecting to the official website and that any information you provide is encrypted and transmitted securely.
- Publications
- Account settings
Preview improvements coming to the PMC website in October 2024. Learn More or Try it out now .
- Advanced Search
- Journal List
- Front Plant Sci
Impact of climate change on agricultural production; Issues, challenges, and opportunities in Asia
Muhammad habib-ur-rahman.
1 Institute of Crop Science and Resource Conservation (INRES), Crop Science Group, University of Bonn, Bonn, Germany
2 Department of Agronomy, MNS-University of Agriculture, Multan, Pakistan
Ashfaq Ahmad
3 Asian Disaster Preparedness Center, Islamabad, Pakistan
4 Department of Agronomy, University of Agriculture Faisalabad, Faisalabad, Pakistan
Muhammad Usama Hasnain
Hesham f. alharby.
5 Department of Biological Sciences, Faculty of Science, King Abdulaziz University, Jeddah, Saudi Arabia
Yahya M. Alzahrani
Atif a. bamagoos, khalid rehman hakeem.
6 Princess Dr. Najla Bint Saud Al-Saud Center for Excellence Research in Biotechnology, King Abdulaziz University, Jeddah, Saudi Arabia
7 Department of Public Health, Daffodil International University, Dhaka, Bangladesh
Saeed Ahmad
8 Institute of Plant Breeding and Biotechnology, MNS-University of Agriculture, Multan, Pakistan
9 Department of Agronomy, The Islamia University, Bahwalpur, Pakistan
Wajid Nasim
Shafaqat ali.
10 Department of Environmental Science and Engineering, Government College University, Faisalabad, Pakistan
Fatma Mansour
11 Department of Economics, Business and Economics Faculty, Siirt University, Siirt, Turkey
Ayman EL Sabagh
12 Department of Agronomy, Faculty of Agriculture, Kafrelsheikh University, Kafrelsheikh, Egypt
13 Department of Field Crops, Faculty of Agriculture, Siirt University, Siirt, Turkey
Agricultural production is under threat due to climate change in food insecure regions, especially in Asian countries. Various climate-driven extremes, i.e., drought, heat waves, erratic and intense rainfall patterns, storms, floods, and emerging insect pests have adversely affected the livelihood of the farmers. Future climatic predictions showed a significant increase in temperature, and erratic rainfall with higher intensity while variability exists in climatic patterns for climate extremes prediction. For mid-century (2040–2069), it is projected that there will be a rise of 2.8°C in maximum temperature and a 2.2°C in minimum temperature in Pakistan. To respond to the adverse effects of climate change scenarios, there is a need to optimize the climate-smart and resilient agricultural practices and technology for sustainable productivity. Therefore, a case study was carried out to quantify climate change effects on rice and wheat crops and to develop adaptation strategies for the rice-wheat cropping system during the mid-century (2040–2069) as these two crops have significant contributions to food production. For the quantification of adverse impacts of climate change in farmer fields, a multidisciplinary approach consisted of five climate models (GCMs), two crop models (DSSAT and APSIM) and an economic model [Trade-off Analysis, Minimum Data Model Approach (TOAMD)] was used in this case study. DSSAT predicted that there would be a yield reduction of 15.2% in rice and 14.1% in wheat and APSIM showed that there would be a yield reduction of 17.2% in rice and 12% in wheat. Adaptation technology, by modification in crop management like sowing time and density, nitrogen, and irrigation application have the potential to enhance the overall productivity and profitability of the rice-wheat cropping system under climate change scenarios. Moreover, this paper reviews current literature regarding adverse climate change impacts on agricultural productivity, associated main issues, challenges, and opportunities for sustainable productivity of agriculture to ensure food security in Asia. Flowing opportunities such as altering sowing time and planting density of crops, crop rotation with legumes, agroforestry, mixed livestock systems, climate resilient plants, livestock and fish breeds, farming of monogastric livestock, early warning systems and decision support systems, carbon sequestration, climate, water, energy, and soil smart technologies, and promotion of biodiversity have the potential to reduce the negative effects of climate change.
Introduction
Asia is the most populous subcontinent in the world (UNO, 2015 ), comprising 4.5 billion people—about 60% of the total world population. Almost 70% of the total population lives in rural areas and 75% of the rural population are poor and most at risk due to climate change, particularly in arid and semi-arid regions (Yadav and Lal, 2018 ; Population of Asia, 2019 ). The population in Asia is projected to reach up to 5.2 billion by 2050, and it is, therefore, challenging to meet the food demands and ensure food security in Asia (Rao et al., 2019 ). In this context, Asia is the region most likely to attribute to population growth rate, and more prone to higher temperatures, drought, flooding, and rising sea level (Guo et al., 2018 ; Hasnat et al., 2019 ). In Asia, diversification in income of small and poor farmers and increasing urbanization is shocking for agricultural productivity. Asia is the home of a third of the world's population and the majority of poor families, most of which are engaged in agriculture (World Bank, 2018 ). We can expect diversification of adverse climate change effects on the agriculture sector due to diversity of farming and cropping systems with dependence on climate. According to the sixth assessment report of IPCC, higher risks of flood and drought make Asian agricultural productivity highly susceptible to changing climate (IPCC, 2019 ). Climate change has already adversely affected economic growth and development in Asia, although there is low emission of greenhouse gasses (GHG) in this region (Gouldson et al., 2016 ; Ahmed et al., 2019a ). Still, China and India are major contributors to global carbon dioxide emission; the share of each Asian country in cumulative global carbon dioxide emission is presented in Figures 1 , ,2. 2 . Although GHGs emission from the agriculture sector is lower than the others, it still has a negative impact. Emission of GHGs from different agricultural components and contribution to emissions can be found in Figure 3 . However, the contribution of Asian countries in GHGs including land use changes and forestry is described in Figure 4 .

Share of each Asian country in cumulative global carbon dioxide emission (1751–2019; Source: OWID based on CDIAC and Global Carbon Project).

Carbon dioxide (CO 2 ) emission from different Asian countries (source: International Energy Statistics https://cdiac.ess-dive.lbl.gov/home.html ; Carbon Dioxide Information Analysis Center, Environmental Sciences Division, Oak Ridge National Laboratory, Tennessee, United States).

Sources of greenhouse gasses (GHGs) emission from different Asian countries with respect to agricultural components (Source: CAIT climate data explorer via . Climate Watch ( https://www.climatewatchdata.org/data-explorer/historical-emissions ).

Total greenhouse gasses (GHGs) emission includes emissions from land use changes and forestry from Asian countries (measured in tons of carbon dioxide equivalents [CO 2 -e] (Source: CAIT climate data explorer via Climate Watch).
Asia is facing alarming challenges due to climate change and variability as illustrated by various climatic models predicting the global mean temperature will increase by 1.5°C between 2030 and 2050 if it continues to increase at the current rate (IPCC, 2019 ). In arid areas of the western part of China, Pakistan, and India, it is also projected that there will be a significant increase in temperature (IPCC, 2019 ). During monsoon season, there would be an increase in erratic rainfall of high intensity across the region. In South and Southeast Asia, there would be an increase in aridity due to a reduction in winter rainfall. Due to climatic abnormalities, there will be a 0.1 m increase in sea level by 2,100 across the globe (IPCC, 2019 ). In Asia, an increase in heat waves, hot and dry days, and erratic and unsure rainfall patterns is projected, while dust storms and tropical cyclones are predicted to be worse in the future (Gouldson et al., 2016 ). Natural disasters are the main reason behind the agricultural productivity (crops and livestock) losses in Asia, including extreme temperature, storms and wildfires (23%), floods (37%), drought (19%), and pest and animal diseases infestation (9%) which accounted for 10 USD billions in amount (FAO, 2015 ). During the last few decades, tropical cyclones in the Pacific have occurred with increased frequency and intensity. South Asia consisted of 262 million malnourished inhabitants, which made South Asia the most food insecure region across the globe (FAO, 2015 ; Rasul et al., 2019 ). In remote dry lands and deserts, the rural population is more vulnerable to climate change due to the scarcity of natural resources.
In Asia, climate variability (temperature and rainfall) and climate-driven extremes (flood, drought, heat stress, cold waves, and storms) have several negative impacts on the agriculture sector (FAO, 2016 ), especially in the cropping system which has a major role in food security, and thus created the food security issues and challenges in Asia (Cai et al., 2016 ; Aryal et al., 2019 ). The rice-wheat cropping system, a major cropping system which fills half of the food demand in Asia, is under threat due to climate change (Ghaffar et al., 2022 ). Climate change adversely affects both the quantity and quality of wheat and rice crops (Din et al., 2022 ; Wasaya et al., 2022 ). For instance, the protein content and grain yield of wheat have been reduced because of the negative impacts of increasing temperature (Asseng et al., 2019 ). The temperature rise has decreased the crop-growing period, and crop evapotranspiration ultimately reduced wheat yield (Azad et al., 2018 ). Adverse impacts of climate change and variability on winter wheat yield in China are attributed to increased average temperature during the growing period (Geng et al., 2019 ). Climate change is also adversely affecting the quality traits especially protein content, and sugars and starch percentages in grains of wheat. Elevated carbon dioxide and high temperatures increase the growth traits while decreasing the protein content in wheat grains (Asseng et al., 2019 ). Similarly, drought stress also reduces the protein content and soluble sugars of the wheat crop (Rakszegi et al., 2019 ; Hussein et al., 2022 ). The decline in the starch content in wheat grains has also been observed under drought stress (Noori and Taliman, 2022 ). Similarly, heat stress also causes a decline in the protein content, soluble sugar, and starch content in wheat grains (Zahra et al., 2021 ; Iqbal et al., 2022 ; Zhao et al., 2022 ). Climate change also negatively affects the quality of wheat products as the rise in temperature causes a reduction in protein content, sugars, and starch. It is assessed that rise in temperature by 1–4°C could decrease the wheat yield up to 17.6% in the Egyptian North Nile Delta (Kheir et al., 2019 ). In China, crop phenology has changed because of both climate variability and crop management practices (Liu et al., 2018 ). Both climate change scenarios and human management practices have adversely affected wheat phenology in India and China (Lv et al., 2013 ; Ren et al., 2019 ). The elevated temperature has increased the infestation of the aphid population on wheat crops and ultimately reduced yield (Tian et al., 2019 ). There is a direct and strong correlation between diseases attached to climate change. For instance, the Fusarium head blight of wheat crops is caused by the Fusarium species and its chances of an attack were increased due to high humidity and hot environment (Shah et al., 2018 ). A similar study has shown a direct interaction between insect pests and diseases and higher temperature and carbon dioxide levels in rice production (Iannella et al., 2021 ; Tan et al., 2021 ; Tonnang et al., 2022 ).
Climate variability has marked several detriments to rice production in Asia. Climate variability has induced flood and drought, which have decreased the rice yield in South Asia and several other parts of Asia (Mottaleb et al., 2017 ). Heat stress, drought, flood, and cyclones have reduced the rice yield in South Asia (Cai et al., 2016 ; Quyen et al., 2018 ; Tariq et al., 2018 ). Thus, climate change-driven extremes, particularly heat and drought stress, have also become a serious threat for sustainable rice production globally (Xu et al., 2021 ). Higher temperatures for a longer period as well as water shortages reduce seed germination which lead to poor stand establishment and seedling vigor (Fahad et al., 2017 ; Liu et al., 2019 ). It has been reported that the exposure of rice crops to high temperatures (38°C day/30°C night) at the grain filling stage led to a reduction in grain weight of rice (Shi et al., 2017 ). Moreover, heat stress also reduces the panicle and spikelet's initiation and ultimately the number of spikelets and grains in the rice production system (Xu et al., 2020 ). Drought stress also adversely affects the reproductive stages and reduces the yield components especially spikelets per panicle, grain size, and grain weight of rice (Raman et al., 2012 ; Kumar et al., 2020 ; Sohag et al., 2020 ). GLAM-Rice model has projected rice yield will decrease ~45% in the 2080's under RCP 8.5 as compared to 1991–2000 in Southeast Asia (Chun et al., 2016 ). On the other hand, climate variability could reduce crop water productivity by 32% under RCP 4.5, or 29% under RCP 8.5 by 2080's in rice crops (Boonwichai et al., 2019 ). In China and Pakistan, high temperature adversely affects the booting and anthesis growth stages of rice ultimately resulting in yield reduction (Zafar et al., 2018 ; Nasir et al., 2020 ). Crop models like DSSAT and APSIM have projected a yield reduction of both rice and wheat crops up to 19 and 12% respectively by 2069 due to a rise of 2.8°C in maximum and 2.2°C in minimum temperature in Pakistan (Ahmad et al., 2019 ).
About 35 million farmers having 3% landholding are projected to convert their source of income (combined crop-livestock production systems) to simply livestock because of the negative impacts of climate change on the quality and quantity of pastures as predicted by future scenarios for 2050 in Asia (Thornton and Herrero, 2010 ). The livestock production sector also contributes 14.5% of global greenhouse emissions and drives climate variability (Downing et al., 2017 ). Directly, there would be higher disease infestation and reduced milk production and fertility rates in livestock because of climate extremes like heat waves (Das, 2018 ; Kumar et al., 2018 ). Indirectly, heat stress will reduce both the quantity and quality of available forage for livestock. Several studies have reported that heat stress reduces the protein and starch content in the grains of maize which is a widely used forage crop (Yang et al., 2018 ; Bheemanahalli et al., 2022 ). Similarly, heat stress also reduces the soluble sugar and protein content in the heat-sensitive cultivars of alfalfa which is also a major forage crop (Wassie et al., 2019 ). In this context, heat stress leads to a reduction in the quality of forage. There would be an increase in demand for livestock products, however, there would be a decrease in livestock heads under future climate scenarios (Downing et al., 2017 ). In Asia, a severe shortage of feed for livestock has imposed horrible effects on the livestock population which has been attributed as the result of extreme rainfall variability and drought conditions (Ma et al., 2018 ).
Timber forests have several significances in Asia, and non-timber forests are also significant sources of food, fiber, and medicines (Chitale et al., 2018 ). Unfortunately, climate change has imposed several negative impacts on forests at various levels in the form of productive traits, depletion of soil resources, carbon dynamics, and vegetation shifting in Asian countries. In India, forests are providing various services in terms of meeting the food demand of 300 million people, the energy demand of people living in rural areas up to 40%, and shelter to one-third of animals (Jhariya et al., 2019 ). In Bangladesh, forests are also vulnerable to climate variability as they are facing the increased risks of fires, rise in sea level, storm surges, coastal erosion, and landslides (Chow et al., 2019 ). Increased extreme drought events with higher frequency, intensity, and duration, and human activities, i.e., afforestation and deforestation, have adversely altered the forest structure (Xu et al., 2018 ). Hence, there is a need to evaluate climate adaptation strategies to restore forests in Asian countries in order to meet increased demands of food, fiber, and medicines. Agroforestry production is also under threat because of adverse climate change impacts such as depletion of natural resources, predominance of insect pests, diseases and unwanted species, increased damage on agriculture and forests, and enhanced food insecurity (De Zoysa and Inoue, 2014 ; Lima et al., 2022 ).
Asia also consists of good quality aquaculture (80% of aquaculture production worldwide) and fisheries (52% of wild caught fish worldwide) which are 77% of the total value addition (Nguyen, 2015 ; Suryadi, 2020 ). In Asia, various climatic extremes such as erratic rainfall, drought, floods, heat stress, salinity, cyclone, ocean acidification, and increased sea level have negatively affected aquaculture (Ahmad et al., 2019 ). For instance, Hilsailisha constituted the largest fishery in Bangladesh, India, and West Bengal and S. Yangi in China have lost their habitat because of climate variability (Jahan et al., 2017 ; Wang et al., 2019a ). Ocean acidification and warming of 1.5°C was closely associated with anthropogenic absorption of CO 2 . Increasing levels of ocean acidity is the main threat to algae and fish. Among various climate driven extremes like drought, flood, and temperature rising, drought is more dangerous as there is not sufficient rainfall especially for aquaculture (Adhikari et al., 2018 ). Similarly, erratic rainfall, irregular rainfall, storms, and temperature variability have posed late maturity in fish for breeding and other various problems (Islam and Haq, 2018 ).
The above-mentioned facts have indicated that agriculture, livestock, forestry, fishery, and aquaculture are under threat in the future and can drastically affect food security in Asia. This paper reviews the climate change and variability impacts on the cropping system (rice and wheat), livestock, forestry, fishery, and aquaculture and their issues, challenges, and opportunities. The objectives of the study are to: (i) Review the climate variability impacts on agriculture, livestock, forestry, fishery, and aquaculture in Asia; (ii) summarize the opportunities (adaptation and mitigation strategies) to minimize the drastic effects of climate variability in Asia; and (iii) evaluate the impact of climate change on rice-wheat farmer fields—A case study of Pakistan.
Impact of climate change and variability on agricultural productivity
Impact of climate change and variability on rice-wheat crops.
In many parts of Asia, a significant reduction in crop productivity is associated with a reduction in timely water and rainfall availability, and erratic and intense rainfall patterns during the last decades (Hussain et al., 2018 ; Aryal et al., 2019 ). Despite the increased crop production owing to the green revolution, there is a big challenge to sustain production and improve food security for poor rural populations in Asia under climate change scenarios (FAO, 2015 ; Ahmad et al., 2019 ). In the least developed countries, damage because of climactic changes may threaten food security and national economic productivity (Myers et al., 2017 ). Yield reductions in different crops (rice, wheat) varied within regions due to variations in climate patterns (Yu et al., 2018 ). CO 2 fertilization can increase crop productivity and balance the drastic effects of higher temperature in C 3 plants (Obermeier et al., 2017 ) but cannot reduce the effect of elevated temperature (Arunrat et al., 2018 ). Crop growth and development have been negatively influenced because of rising temperatures and rainfall variability (Rezaei et al., 2018 ; Asseng et al., 2019 ).
Rice and wheat are major contributors to food security in Asia. There is a big challenge to increase wheat production by 60% by 2050 to meet ever-enhancing food demands (Rezaei et al., 2018 ). In arid to semi-arid regions, declined crop productivity is attributed to an increase in temperature at lower latitudes. In China, drought and flood have reduced the rice, wheat, and maize yields and it is projected that these issues will affect crop productivity more significantly in the future (Chen et al., 2018 ). Rice is sensitive to a gradual rise in night temperature causing yield and biomass to reduce by 16–52% if the temperature increase is 2°C above the critical temperature of 24°C (Yang et al., 2017 ). In Asia, semi-arid to arid regions are under threat and are already facing the problem of drought stress and low productivity. The quality of wheat produce (protein content, sugars, and starch) and grain yield have reduced because of the negative impacts of increasing temperature and erratic rainfall with high intensity (Yang et al., 2017 ). In the Egyptian North Nile Delta (up to 17.6%), India, and China, the climate variability has decreased wheat yield significantly which is attributed to a rise in temperature, erratic rainfall and increasing insect pest infestation (Arunrat et al., 2018 ; Shah et al., 2018 ; Aryal et al., 2019 ; Kheir et al., 2019 ). In South Asia, rice yield in rain-fed areas has already decreased and it might reduce by 14% under the RCP 4.5 scenario while 10% under the RCP 8.5 scenario by 2080 (Chun et al., 2016 ). High temperature and drought have decreased the rice yield because of their adverse impacts on the booting and anthesis stage in Asia, especially in Pakistan and China (Zafar et al., 2018 ; Ahmad et al., 2019 ). Similarly, heat stress is a major threat to rice as it decreases the productive tillers, shrinkage of grains, and ultimately grain yield of rice (Wang et al., 2019b ). In Asia, climate change would affect upland rice (10 m ha) and rain-fed lowland rice (>13 million hectares). The projected production of rice and wheat crops by 2030 is presented in Table 1 .
Productivity shock due to climate change and variability on rice and wheat crop production by 2030.
Source: Gouldson et al. ( 2016 ), Asseng et al. ( 2019 ), Chow et al. ( 2019 ), Degani et al. ( 2019 ), Sanz-Cobena et al. ( 2019 ), and Suryadi ( 2020 ).
Minus sign (-) indicates the decrease in productivity while positive sign (+) indicates increase in productivity.
Impact of climate change and variability on livestock
In arid to semi-arid regions, the livestock sector is highly susceptible to increased temperature and reduced precipitation (Downing et al., 2017 ; Balamurugan et al., 2018 ). A temperature range of 10–30°C is comfortable for domestic livestock with a 3–5% reduction in animal feed intake with each degree rise in temperature. Similarly, the lower temperature would increase the requirement feed up to 59%. Moreover, drought and heat stress would drastically affect livestock production under climate change scenarios (Habeeb et al., 2018 ). Climate variability affects the occurrence and transmission of several diseases in livestock. For instance, Rift Valley Fever (RVF) due to an increase in precipitation, and tick-borne diseases (TBDs) due to a rise in temperature, have become epidemics for sheep, goats, cattle, buffalo, and camels (Bett et al., 2019 ). Different breeds of livestock show different responses to higher temperature and scarcity of water. In India, thermal stress has negative impacts on the reproduction traits of animals and ultimately poor growth and high mortality rates of poultry (Balamurugan et al., 2018 ; Chen et al., 2021 ; van Wettere et al., 2021 ). In dry regions of Asia, extreme variability in rainfall and drought stress would cause severe feed scarcity (Arunrat et al., 2018 ). It has been revealed that a high concentration of CO 2 reduces the quality of fodder like the reduction in protein, iron, zinc, and vitamins B1, B2, B5, and B9 (Ebi and Loladze, 2019 ). Future climate scenarios show that the pastures, grasslands, feedstuff quality and quantity, as well as biodiversity would be highly affected. Livestock productivity under future climate scenarios would affect the sustainability of rangelands, their carrying capacity and ecosystem buffering capacity, and grazing management, as well as the alteration in feed choice and emission of greenhouse gases (Nguyen et al., 2019 ).
Impact of climate change on forest
Climate variability has posed several negative impacts on forests including variations in productive traits, carbon dynamics, and vegetation shift, as well as the exhaustion of soil resources along with drought and heat stress in South Asian countries (Jhariya et al., 2019 ; Zhu et al., 2021 ). In Bangladesh, forests are vulnerable to climate variability due to increased risks of fires, rise in sea level, storm surges, coastal erosion and landslides, and ultimately reduction in forest area (Chow et al., 2019 ). Biodiversity protection, carbon sequestration, food, fiber, improvement in water quality, and medicinal products are considered major facilities provided by forests (Chitale et al., 2018 ). In contrast, trait-climate relationships and environmental conditions have drastically influenced structure, distribution, and forest ecology (Keenan, 2015 ). Higher rates of tree mortality and die-off have been induced in forest trees because of high temperature and often-dry events (Allen et al., 2015 ; Greenwood et al., 2017 ; Zhu et al., 2021 ). For instance, trees Sal, pine trees, and Garjan have been threatened by climate-driven continuing forest clearing, habitat alteration, and drought in South Asian countries (Wang et al., 2019). An increase in temperature and CO 2 fertilization has increased insect pest infestation for forest trees in North China (Bao et al., 2019 ). As rising temperature, elevated carbon dioxide (CO 2 ), and fluctuating precipitating patterns lead to the rapid development of insect pests and ultimately more progeny will attack forest trees (Raza et al., 2015 ). Hence, there is a need to develop adaptation strategies to restore forests to meet the increasing demand for food, fiber, and medicines in Asia.
Impact of climate change on aquaculture and fisheries
There is a vast difference in response to climate change scenarios of aquaculture in comparison to terrestrial agriculture due to greater control levels over the production environment under terrestrial agriculture (Ottaviani et al., 2017 ; Southgate and Lucas, 2019 ). Climatic-driven extremes such as drought, flood, cyclones, global warming, ocean acidification, irregular and erratic rainfall, salinity, and sea level rise have negatively affected aquaculture in South Asia (Islam and Haq, 2018 ; Ahmad et al., 2019 ). In Asia, various species such as Hilsa and algae have lost their habitats due to ocean acidification and temperature rise (Jahan et al., 2017 ). Increased water temperature and acidification of terrestrial agriculture have become dangerous for coral reefs and an increase in average temperature by 1°C for four successive weeks can cause bleaching of coral reefs in India and other parts of Asia (Hilmi et al., 2019 ; Lam et al., 2019 ). Ocean warming has caused severe damage to China's marine fisheries (Liang et al., 2018 ). In Pakistan, aquaculture and fisheries have lost their habitat quality, especially fish breeding grounds because of high cyclonic activity, sea level rise, temperature variability, and increased invasion of saline water near Indus Delta (Ali et al., 2019 ). It is revealed that freshwater and brackish aquaculture is susceptible to the negative effects of climate variability in several countries of Asia (Handisyde et al., 2017 ). It is also evaluated that extreme climate variability has deep impacts on wetlands and ultimately aquaculture in India (Sarkar and Borah, 2018 ).
Climate variability and change impact assessment
Agriculture has a complex structure and interactions with different components, which will make it uncertain in a future climate that is a serious risk to food security in the region. Consequently, it is essential to assess the negative impacts of climate change on agricultural productivity and develop adaptive strategies to combat climate change. Simulation models such as General Circulation Models (GCMs) and Representative Concentration Pathways (RCPs) are being used worldwide for the quantification of the negative effects of climate change on agriculture and are supporting the generation of future weather data (Rahman et al., 2018 ). Primary tools are also available that can estimate the negative impacts of changing climate on crop productivity, crucial for both availability and access to food. Crop models have the potential to describe the inside processes of crops by considering the temperature rise and elevated CO 2 at critical crop growth stages (Challinor et al., 2018 ). There are no advanced methods and technologies available to see the impact of climate variability and change on the production of livestock and crops other than the modeling approach (Asseng et al., 2014 ). There are also modeling tools available, and being used across the world, to quantify the impacts of climate change and variability on crops and livestock production (Ewert et al., 2015 ; Hoogenboom et al., 2015 ; Rahman et al., 2019 ). We decided to quantify the impacts of future climate on farmer's livelihood to study the complete agricultural system by adopting the comprehensive methodology of climate, crop, and economic modeling (RAPs) approaches and found the agricultural model inter-comparison and improvement project (AgMIP) as the best approach.
A case study—Agricultural model inter-comparison and improvement project
Impact of climate change on the productivity of rice and wheat crops.
Department for International Development (DFID) developed the Agricultural Model Inter-comparison and Improvement Project (Rosenzweig et al., 2013 ) which is an international collaborative effort to deeply investigate the influences of climate variability and change on crops' productivity in different cropping zones/systems across the world and in Pakistan. The mission of AgMIP is to improve the scientific capabilities for assessing the impact of climate variability on the agricultural production system and develop site-specific adaptation strategies to ensure food security at local to global scales. The review discussed above indicated that the agriculture sector is the most vulnerable due to climatic variability and change. Crop production is under threat in Asian countries—predominantly in developing countries. For instance, Pakistan is also highly vulnerable due to its geographical location with arid to semi-arid environmental conditions (Nasi et al., 2018 ; Ullah et al., 2019 ; Ghaffar et al., 2022 ). There would be impacts that are more adverse in arid and semi-arid regions in comparison to humid regions because of climate change and variability (Nasi et al., 2018 ; Ali et al., 2019 ). Future climate scenarios have uncertainty and the projected scenario of climate, especially precipitation, did not coincide with the production technology of crops (Rahman et al., 2018 ). Floods and drought are anticipated more due to variations in rainfall patterns, and dry seasons are expected to get drier in future. Developing regions of the globe are more sensitive to climate variability and change as these regions implement old technologies whereas developed regions can mediate climate-driven extremes through the implementation of modern technologies (Lybbert and Sumner, 2012 ). The extent of climate change and variability hazards in Pakistan is massive and may be further shocking in the future. Therefore, it is a matter of time to compute climate variability, impacts on crop production, and develop sustainable adaptation strategies to cope with the negative impact of climate change using AgMIP standards and protocols (AgMIP). The main objective is to formulate adaptation strategies to contradict potential climate change effects and support the livelihood of smallholder farmers in the identified area and circulate this particular information to farmers, extension workers, and policy-makers. Sialkot, Sheikhupura, Nankana sahib, Hafizabad, and Gujranwala are considered the hub of the rice-wheat cropping system (Ghaffar et al., 2022 ), with an area of 1.1 million hectares. The rice-wheat cropping system is a food basket and its sustainable productivity in future climates will ensure food security in the country and generally overall in the region.
Methodology of the case study
Field data collection.
Field data included the experimental trials and socio-economic data of 155 successive farmers' farms collected during an extensive survey of rice-wheat cropping zone from five-selected districts ( Figure 5 ). From each district, randomly two villages were selected from each division, randomly 30 respondents and 15 farms of true representation of the farming population from each village considered. Crop management data included all agronomic practices from sowing to harvesting such as planting time, planting density, fertilizers amount and organic matter amendment, irrigation amount and intervals, cultural operations, grain yield, and biomass production collected for both crops, rice and wheat, and overall, for all systems. Farm data for the rice-wheat cropping system were analyzed with crop and economic models to see the impact of climate variability on crop production.

Map of study location/sites in rice-wheat cropping zone of Pakistan.
Historic and future climatic data
Daily historic data was collected from the Pakistan Meteorological Department (PMD) for all study locations. The quality of observed weather data was checked following the protocol of the Agricultural Model Inter-comparison and Improvement Project (AgMIP) protocols (AgMIP, 2013 ). Station-based downscaling was performed with historic weather data from all study sites/locations in the rice-wheat cropping zone. For the zone/region, five GCMs (CCSM4, GFDL-ESM2M, MIROC5, HadGEM2-ES, and MPI-ESM-MR) of the latest CMIP5 family were engaged for the generation of climate projections for the mid-century period using the RCP 8.5 concentration scenario, and using the protocols and methodology developed by AgMIP (Ruane et al., 2013 , 2015 ; Rahman et al., 2018 ). GCMs were selected on the basis of different factors such as better performance in monsoon seasons, the record of accomplishment of publications, and the status of the model-developing institute. Under the RCP 8.5 scenario, an indication of warming ranges 2–3°C might be expected in all selected districts for the five CMIP5, GCMs in comparison to the baseline between the periods of 2040–2069. However, there is no uniform warming recorded under all 5 CMIP5 GCMs. For instance, CCSM4 and GFDL-ESM-2M showed uniform increased temperatures during April and September months. The outputs of the GCMs indicated large variability in the estimated values of precipitation. The HadGEM2-ES and GFDL-ESM2M projected mean of 200 and 100 mm between times 2040–2069, respectively. On average, a minor rise in annual rainfall (mm) is indicated by five GCMs in comparison to the baseline.
Crop models (DSSAT and APSIM)
To understand the agronomic practices and the impact of climate variability on the development and growth of plants, crop simulation models like DSSATv4.6 (Hoogenboom et al., 2015 , 2019 ) and APSIMv7.5 (Keating et al., 2003 ) were applied. Three field trials were conducted on rice and wheat crops during two growing seasons, to collect the data like phenology, crop growth (leaf area index, biomass accumulation), development, yield, and agronomic management data by following the standard procedure and protocols. Crop models are calibrated with experimental field data (phenology, growth, and yield data) under local environmental conditions by using soil and weather data. Crop models were further validated with farmers' field data of rice and wheat crops. Climate variability impact on both crops was assessed with historic data (baseline) and future climate data of mid-century in this region.
Tradeoff analysis model for multi-dimensional impact assessment
For the analysis of climate change impact socio-economic indicators, version 6.0.1 of the Tradeoff Analysis Model for Multi-Dimensional Impact Assessment (TOA-MD) Beta was employed (Antle, 2011 ; Antle et al., 2014 ). It is an economical and standard model employed for the analysis of technology adoption impact assessment and ecosystem services. Schematically illustrated, showing connections between the different models and the points of contact between them in terms of input-output in a different climate, crop and economic models and climate analysis is shown in Figure 6 . Various factors that may affect the anticipated values of the production system are technology, physical environment, social environment, and representative agricultural pathways (RAPs), hence it is necessary to distinguish these factors (Rosenzweig et al., 2013 ). RAPs are the qualitative storylines that can be translated into model parameters such as farm and household size, practices, policy, and production costs. For climate impact assessment, the dimensionality of the analysis is the main threat in scenario design. Farmers employ different systems for operating a base technology. For instance, system 1 included base climate, in system 2, farmers use hybrid climate, and in system 3, farmers use perturbed climate to cope with future climate with adaptation technology. The analysis gave the answer to three core questions (Rosenzweig et al., 2013 ). First, without the application RAPs of the core question, one-climate change impact assessments (CC-IA) were formulated. Second, analysis was again executed for examining the negative effects of climate change on future production systems. Third, analysis was executed for future adapted production systems through RAPs and adaptations. Two crop models, i.e., DSSAT and APSIM, outputs were used as the inputs of TOA-MD. Different statistical analyses like root mean square error (RMSE), mean percentage difference (MPD) d-stat, percent difference (PD), and coefficient of determination (R2) were used to check the accuracy of models.

Schematic illustration showing connections between the different models (climate, crop, and economic) and the points of contact between them in terms of input-output and climate analysis.
Farmers field data validation
Crop model simulation results regarding calibration and validation of both crops (rice and wheat) were in good agreement with the field experimental data. Both models were further validated using farmers' field data of rice and wheat crops in rice-wheat cropping zone after getting robust genetic coefficients. Model validation results of 155 farmers of rice and wheat crops indicated the good accuracy of both models (DSSAT, APSIM) and have a good range of statistical indices. Both of these crop models showed an improved ratio between projected and observed rice yield in farmers' fields with RMSE 409 and 440 kg ha −1 and d-stat 0.80 and 0.78, respectively. Similarly, the performance of models DSSAT and APSIM for a yield of wheat was also predicted with RMSE of 436 and 592 kg ha −1 and d-stat of 0.87, respectively.
Quantification of climate change impact by crop models
Climate change impact assessment results in the rice-wheat cropping zone of 155 farms indicated that yield reduction varied due to differences in GCM's behavior and variability in climatic patterns. It is predicted that mean rice yield reduction would be up to 15 and 17% for DSSAT and APSIM respectively during mid-century while yield reduction variation among GCMs are presented in Figure 7 . Rice indicated a yield decline ranging from 14.5 to 19.3% for the case of APSIM while mean yield reduction of the rice crop was between 8 and 30% with DSSAT. Reduction in production of wheat varied among GCMs as well as an overall reduction in yield in rice-wheat cropping systems. For wheat, with DSSAT would be a 14% reduction whereas for APSIM, the reduction would be 12%. GCMs reduction in wheat yield for midcentury (2040–2069) is shown in Figure 8 . Reduction in wheat yield for all 5 GCMs was from 10.6 to 12.3% in the case of APSIM while mean reduction in wheat yield was between 6.2 and 19%. As rice is a summer crop where the temperature is already high and, according to climate change scenarios, there is an increase in both maximum and minimum temperature, an increase in minimum temperature leads to more reduction in yield as compared to wheat being a winter season crop. It was hypothesized that the increase in night temperature (minimum temperature) leading to more losses in the summer season may be due to high temperature, particularly at anthesis and grain formation stages in rice crops, as it is already an irrigated crop and rainfall variability (more rainfall) cannot reduce the effect of high temperature in the rice yield as compared to the wheat crop.

Reduction in rice yield of APSIM and DSSAT models for 155 farms; variation with 5-GCMs in rice-wheat cropping system of Punjab-Pakistan.

Reduction in wheat yield of APSIM and DSSAT models for 155 farms; variation with 5-GCMs in rice-wheat cropping system of Punjab-Pakistan.
Climate change economic impact assessment and adaptations
Sensitivity of current agricultural production systems to climate change.
Climate change is damaging the present vulnerabilities of poor small farmers as their livelihood depends directly on agriculture. Noting various impacts of future climate (2040–2069) on a current production system (current technologies), we examine the vulnerability of the current production system used for the assessment of the adverse impacts of climate change on crop productivity and other socio-economic factors. Climate change impacts possible outcomes for five GCMs based on the estimation of yield generated by two crop models presented in Table 2 . In Table 3 , and the grain losses and net impacts as a percentage of average net returns for the first core question are given for each GCM. The analysis clearly shows the observed values of the mean yield of wheat and rice, which are estimated to be 18,915 kg and 18,349 kg/ farm respectively in the projected area. For all GCMs, observed average milk production was 3,267 liters per farm with a 12% average decline in yield found under livestock production. Losses were about 69–83% and from 72 to 76% for DSSAT and APSIM respectively as predicted by TOA-MD analysis because of the adverse effects of climate change situations. For DSSAT, percentage losses and gains in average net farm returns were from 13 to 15% and 23 to 30%, respectively. While gains were 14–15% and losses were from 25 to 27%, respectively for APSIM. Without adverse impacts of climate change, a net income of Rs. 0.54 per farm pragmatic was predicted by DSSAT and APSIM. However, DSSAT predicted Rs. 0.42–0.48 M per farm and APSIM predicted Rs. 0.45–0.47 M net income per farm under climate change for all GCMs. An increase in the poverty rate in climate change situations would be 33–38% for DSSAT and it would be 35–37% for APSIM, respectively while the rate of poverty with no adverse impacts of climate change would be 29%.
Relative yield summary of crop models.
r = ∑s2/∑s1, ∑s2, Time averaged mean of simulated future yield; ∑s1, Time averaged mean of simulated past yield.
Aggregated gains and losses with CCSM4 GCM (without adaptation and with trend) of DSSAT and APSIM.
Impacts of climate change on future agricultural production systems
In regard to the second core question, a comparison of system 1 (current climate and future production system) with system 2 (future climate and future production system in mid-century) was analyzed with the aid of TOA-MD using 5 GCMs. Mean wheat and rice yield reduction for DSSAT was from 6.2 to 19% and 8 to 30% respectively, and APSIM indicated a decline ranging from 10.6 to 12.3% and 14 to 19%, respectively. For all analyses of Q2, the projected mean yield was 25,073 kg per farm under rice production. While in the case of livestock for all analyses, the mean projected milk production was 3,267 L/farm with its mean decline in yield estimated to be about 12%. Percentage losses for DSSAT and APSIM would fluctuate between 57 and 70% and from 61 to 71%, respectively for all five GCMs.
Mean net farm returns for gains and losses, as a percentage for DSSAT would be 11–13% and from −16 to −22%, respectively. While the percentage of gains and losses would be between 10 and 15% and −17% and −19% in the case of APSIM, respectively. DSSAT predicted Rs. 89–100 thousand per person while APSIM predicted Rs. 93–97 thousand per person per capita income in changing climatic scenarios. For both crop models, the poverty rate will be 16% without climate change. While poverty rates will be from 17 to 19% in the case of DSSAT and ranging from 18 to 19% for APSIM with climate change ( Table 3 ).
Evaluation of potential adaptation strategies and representative agricultural pathways
Adaptation technologies for rice and wheat crops ( Table 4 ) are used in crop growth models and economic TOA-MD model analysis ( Table 5 ) for simulating the sound effects of prospective adaptation strategies on both adapters and non-adapters distribution. This TOA-MD analysis compared “system 1” (incorporating RAPs) and “system 2” (incorporating RAPs and adapted technology) for the rice-wheat system in the mid-century based on crop models DSSAT and APSIM using 5 GCMs. The mean yield change of wheat and rice crops was from 60 to 72% for DSSAT and 70 to 80% for APSIM respectively, wheat crop indicated a change that ranges from 80 to 89% and 62 to 84% for all five GCMs ( Figure 9 ). Under livestock production, the estimated average production of milk exclusive of adaptation was 3,593 liters/farm for all analyses and for all cases indicates a 42% increase in average yield. The percentage of adopters due to adaptation technologies for DSSAT and APSIM in rice-wheat cropping systems would be between 92 and 93% and 93 and 94%, respectively. For DSSAT and APSIM estimated per head income with adaptation cases will be from Rs. 89 to 100 and 93 to 97 thousand and from Rs. 156 to 174 and 166 to 181 thousand per head, respectively in a year. Without and with adaptation, poverty would range between 17 and 19% and 12 and 13% respectively, for DSSAT and from 18 to 19% and 12 to 13%, respectively for APSIM ( Table 6 ). Climatic changes in the rice-wheat cropping areas of Punjab province will have less impact on the future systems after implementing the adaptation strategies, with a large and significant impact imposed by these adaptations.
Adaptation technology related to crop management used for crop models (DSSAT and PSIM) to cope with the negative impacts of climate change during mid-century (2040–2069).
Percentage change (% change) shows the percentage of farmers using the crop management practices related to crop models to reduce the adverse effects of climate change.
Adaptation technology related to socioeconomic used for crop models (DSSAT and APSIM) to cope with the negative impacts of climate change during mid-century (2040–2069).
Percentage change (% change) shows the percentage of farmers using the socioeconomic technology related to crop models to reduce the adverse effects of climate change.

Distribution of adopters and non-adopters for all 5 GCMs (with adaptation and with trend). The percentage of adopters due to adaptation technologies for DSSAT and APSIM in rice-wheat cropping system would be between 92 and 93% and 93 and 94%, respectively. For DSSAT and APSIM estimated per head income with adaptation cases will be from Rs. 89 to 100 and 93 to 97 thousand and from Rs. 156 to 174 and 166 to 181 thousand per head respectively in a year.
Projected adoption of adaptation package used in crop models for CCSM4 GCM during mid-century.
Opportunities in the era of climate change for agriculture
Scope of adaptation and mitigation strategies for sustainable agricultural production.
It is essential to assess the impact of climate variability on agricultural productivity and develop adaptation strategies/technology to cope with the negative effects to ensure sustainable production. The hazardous climate change effects can be reduced by adapting climate-smart and resilient agricultural practices, which will ensure food security and sustainable agricultural production (Zafar et al., 2018 ; Ahmad et al., 2019 ; Ahmed et al., 2019b ). Adaptation is the best way to handle climate variability and change as it has the potential to minimize hazardous climate change effects for sustainable production (IPCC, 2019 ). Innovative technologies and defensive adaptation can reduce the uncertain and harmful effects of climate on agricultural productivity.
Therefore, to survive the harmful climate change effects, the development and implementation of adaptation strategies are crucial. In developing countries, poverty, food insecurity and declined agricultural productivity are common issues, which indicate the need for mitigation and adaptation measures to sustain productivity (Clair and Lynch, 2010 ; Lybbert and Sumner, 2012 ; Mbow et al., 2014 ). At the national and regional level, the insurance of food security is the major criterion for the effectiveness of mitigation and adaptation. Integration of adaptation and mitigation strategies is a great challenge to promote sustainability and productivity. Climate resilient agricultural production systems can be developed and diversified with the integration of land, water, forest biodiversity, livestock, and aquaculture (Hanjra and Qureshi, 2010 ; Meena et al., 2019 ). Summary and overview of all below discussed potential opportunities are presented in Figure 10 .

Overview of opportunities including adaptations and mitigations strategies for sustainable agriculture production system in Asia.
Reduction in GHGs emission
Reduction in GHGs emissions from agriculture under marginal conditions and production of more food are the major challenges for the development of adaptation and mitigation measures (Smith and Olesen, 2010 ; Garnett, 2011 ; Fujimori et al., 2021 ). Similarly, it is an immediate need to control such practices in agriculture which lead to GHGs emissions, i.e., N 2 O emissions from the application of chemical fertilizers, and CH 4 emissions from livestock and rice production systems (Herrero et al., 2016 ; Allen et al., 2020 ). Similarly, alternate wetting and drying and rice intensification are important to reduce the GHGs emission from rice crops (Nasir et al., 2020 ). Carbon can be restored in soil by minimizing the tillage, reducing soil erosions, managing the acidity of the soil, and implementing crop rotation. By increasing grazing duration and rotational grazing of pastureland, sequestration of carbon can be achieved (Runkle et al., 2018 ). About 0.15 gigatonnes of CO 2 equal to the amount of CO 2 produced in 1 year globally, can be sequestered by adopting appropriate grazing measures (Henderson et al., 2015 ). Development of climate-resilient breeds of animals and plants with higher growth rates and lower GHGs emissions should be developed to survive under harsh climatic conditions. Focus further on innovative research and development for the development of climate-resilient breeds, especially for livestock (Thornton and Herrero, 2010 ; Henry et al., 2012 ; Phand and Pankaj, 2021 ).
Application of ICT and decision support system
To mitigate and adapt to the drastic effects of climate variability and change, information and communication technologies (ICTs) can also play a significant role by promoting green technologies and less energy-consuming technology (Zanamwe and Okunoye, 2013 ; Shafiq et al., 2014 ; Nizam et al., 2020 ). Timely provision of information from early warning systems (EWS) and automatic weather stations (AWS) on drought, floods, seasonal variability, and changing rainfall patterns can provide early warning about natural disasters and preventive measures (Meera et al., 2012 ; Imam et al., 2017 ), and it can also support farmers' efforts to minimize harmful effects on the ecosystems. Geographical information systems (GIS), wireless sensor networks (WSN), mobile technology (MT), web-based applications, satellite technology and UAV can be used to mitigate and adapt to the adverse effects of climate change (Kalas, 2009 ; Karanasios, 2011 ). Application of different climate, crop, and economic models may also help reduce the adverse effects of climate variability and change on crop production (Hoogenboom et al., 2011 , 2015 , 2019 ; Ewert et al., 2015 ).
Crop management and cropping system adaptations
Adaptation strategies have the potential to minimize the negative effect of climate variability by conserving water through changes in irrigation amount, timely application of irrigation water, and reliable water harvesting and conservation techniques (Zanamwe and Okunoye, 2013 ; Paricha et al., 2017 ). Crop-specific management practices like altering the sowing times (Meena et al., 2019 ), crop rotation, intercropping (Hassen et al., 2017 ; Moreira et al., 2018 ), and crop diversification and intensification have a significant positive contribution as adaptation strategies (Hisano et al., 2018 ; Degani et al., 2019 ). Meanwhile, replacement of fossil fuels by introducing new energy crops for sustainable production (Ruane et al., 2013 ) is also crucial for the sustainability of the system. Different kinds of adaptation actions (soil, water, and crop conservation, and well farm management) should be adapted in case of long-term increasing climate change and variability (Williams et al., 2019 ). Similarly, alteration in input use, changing fertilizer rates for increasing the quantity and quality of the produce, and introduction of drought resistant cultivars are some of the crucial adaptation approaches for sustainable production. Therefore, under uncertain environmental conditions, to ensure sustainable productivity, crops having climatic resilient genetic traits should also be introduced (Bailey-Serres et al., 2018 ; Raman et al., 2019 ). Similarly, to ensure the sound livelihood of farmers, it is important to develop resilient crop management as well as risk mitigation strategies.
Opportunities for a sustainable livestock production system
The integration of crop production, rearing of livestock and combined use of rice fields for both rice and fish production lead to enhancing the farmers' income through diversified farming (Alexander et al., 2018 ; Poonam et al., 2019 ). Similarly, variations in pasture rates and their rotation, alteration in grazing times, animal and forage species variation, and combination production of both crops and livestock are the activities related to livestock adaptation strategies (Kurukulasuriya and Rosenthal, 2003 ; Havlik et al., 2013 ). Under changing climate scenarios, sustainable production of livestock should coincide with supplementary feeds, management of livestock with a balanced diet, improved waste management methods, and integration with agroforestry (Thornton and Herrero, 2010 ; Renaudeau et al., 2012 ).
Carbon sequestration and soil management
Selection of more drought-resilient genotypes and combined plantation of hardwood and softwood species (Douglas-fir to species) are considered adaptive changes in forest management under future climate change scenarios (Kolstrom et al., 2011 ; Hashida and Lewis, 2019 ). Similarly, timber growth and harvesting patterns should be linked with rotation periods, and plantation in landscape patterns to reduce shifting and fire of forest tree species under climate-smart conditions for forest management to increase rural families' income for a sustainable agricultural ecosystem (Scherr et al., 2012 ). Although, conventional mitigation methods for the agriculture sector have a pivotal role in forest related strategies, some important measures are also included in which afforestation and reforestation should be increased but degradation and deforestation should be reduced and carbon sequestration can be increased (Spittlehouse, 2005 ; Seddon et al., 2018 ; Arehart et al., 2021 ). Carbon stock enhanced the carbon density of forest and wood products through longer rotation lengths and sustainable forest management (Rana et al., 2017 ; Sangareswari et al., 2018 ). Climate change impacts are reduced through adaptation strategies in agroforestry including tree cover outside the forests, increasing forest carbon stocks, conserving biodiversity, and reducing risks by maintaining soil health sustainability (Mbow et al., 2014 ; Dubey et al., 2019 ). Similarly, climate-smart soil management practices like reduction in grazing intensity, rotation-wise grazing, the inclusion of cover and legumes crops, agroforestry and conservation tillage, and organic amendments should also be promoted to enhance the carbon and nitrogen stocks in soil (Lal, 2007 ; Pineiro et al., 2010 ; Xiong et al., 2016 ; Garcia-Franco et al., 2018 ).
Opportunities for fisheries and aquaculture
Sustainable economic productivity of fisheries and aquaculture requires the adaptation of specific strategies, which leads to minimizing the risks at a small scale (Hanich et al., 2018 ). Therefore, to build up the adaptive capacity of poor rural farmers, measures should be carried out by identifying those areas where local production gets a positive response from variations in climatic conditions (Dagar and Minhas, 2016 ; Karmakar et al., 2018 ). Meanwhile, the need to build the climate-smart capacity of rural populations and other regions to mitigate the harmful impacts of climate change should be recognized. In areas which have flooded conditions and surplus water, the integration of aquaculture with agriculture in these areas provides greater advantages to saline soils through newly adapted aquaculture strategies, i.e, agroforestry (Ahmed et al., 2014 ; Dagar and Yadav, 2017 ; Suryadi, 2020 ). To enhance the food security and living standards of poor rural families, aquaculture and artificial stocking engage the water storage and irrigation structure (Prein, 2002 ; Ogello et al., 2013 ). In Asia, rice productivity is increased by providing nutrients by adapting rice-fish culture in which fish concertedly consume the rice stem borer (Poonam et al., 2019 ). Food productivity can be enhanced by the integration of pond fish culture with crop-livestock systems because it includes the utilization of residues from different systems (Prein, 2002 ; Ahmed et al., 2014 ; Dagar and Yadav, 2017 ; Garlock et al., 2022 ). It is important to compete with future challenges in the system by developing new strains which withstand high levels of salinity and poorer quality of water (Kataria and Verma, 2018 ; Lam et al., 2019 ).
Globally, and particularly in developing nations, variability in climatic patterns due to increased anthropogenic activity has become clear. Asia may face many problems because of changing climate, particularly in South Asian countries due to greater population, geographical location, and undeveloped technologies. The increased seasonal temperature would affect agricultural productivity adversely. Crop growth models with the assistance of climatic and economic models are helpful tools to predict climate change impacts and to formulate adaptation strategies. To respond to the adverse effects of climate change, sustainable productivity under climate-smart and resilient agriculture would be achieved by developing adaptation and mitigation strategies. AgMIP-Pakistan is a good specimen of climate-smart agriculture that would ensure crop productivity in changing climate. It is a multi-disciplinary plan of study for climate change impact assessment and development of the site and crop-specific adaptation technology to ensure food security. Adaptation technology, by modifications in crop management like sowing time and density, and nitrogen and irrigation application has the potential to enhance the overall productivity and profitability under climate change scenarios. The adaptive technology of the rice-wheat cropping system can be implemented in other regions in Asia with similar environmental conditions for sustainable crop production to ensure food security. Early warning systems and trans-disciplinary research across countries are needed to alleviate the harmful effects of climate change in vulnerable regions of Asia. Opportunities as discussed have the potential to minimize the negative effect of climate variability and change. This may include the promotion of agroforestry and mixed livestock and cropping systems, climate-smart water, soil, and energy-related technologies, climate resilient breeds for crops and livestock, and carbon sequestration to help enhance production under climate change. Similarly, the application of ICT-based technologies, EWS, AWS, and decision support systems for decision-making, precision water and nutrient management technologies, and crop insurance may be helpful for sustainable production and food security under climate change.
Author contributions
AA, MH-u-R, and AR: conceptualization, validation, and formal analysis. MH-u-R, SAh, AB, WN, AE, HA, KH, AA, FM, YA, and MH: methodology, editing, supervision, and project administration. Initial draft was prepared by MH-u-R and improved and read by all co-authors. All authors contributed to the article and approved the submitted version.
This research funded by the Deputyship for Research and Innovation, Ministry of Education in Saudi Arabia under grant number (IFPRP: 530-130-1442).
Conflict of interest
The authors declare that the research was conducted in the absence of any commercial or financial relationships that could be construed as a potential conflict of interest.
Publisher's note
All claims expressed in this article are solely those of the authors and do not necessarily represent those of their affiliated organizations, or those of the publisher, the editors and the reviewers. Any product that may be evaluated in this article, or claim that may be made by its manufacturer, is not guaranteed or endorsed by the publisher.
Acknowledgments
The authors extend their appreciation to Deputyship for Research & Innovation, Ministry of Education in Saudi Arabia for funding this research work through the project number (IFPRP: 530-130-1442) and King Abdulaziz University, DSR, Jeddah, Saudi Arabia.
- Adhikari S., Keshav C. A., Barlaya G., Rathod R., Mandal R. N., Ikmail S., et al.. (2018). Adaptation and mitigation strategies of climate change impact in freshwater aquaculture in some states of India . J. Fisheries Sci . 12 , 16–21. 10.21767/1307-234X.1000142 [ CrossRef ] [ Google Scholar ]
- AgMIP (2013). The Coordinated Climate-Crop Modeling Project C3MP: An Initiative of the Agricultural Model Inter Comparison and Improvement Project. C3 MP Protocols and Procedures . London. [ Google Scholar ]
- Ahmad S., Abbas G., Ahmed M., Fatima Z., Anjum M. A., Rasul G., et al.. (2019). Climate warming and management impact on the change of phenology of the rice-wheat cropping system in Punjab, Pakistan . Field Crops Res . 230 , 46–61. 10.1016/j.fcr.2018.10.008 [ CrossRef ] [ Google Scholar ]
- Ahmed A. U., Appadurai A. N., Neelormi S. (2019a). Status of climate change adaptation in South Asia region . Status Climate Change Adapt. Asia Pacific 18 , 125–152. 10.1007/978-3-319-99347-8_7 [ CrossRef ] [ Google Scholar ]
- Ahmed N., Thompson S., Glaser M. (2019b). Global aquaculture productivity, environmental sustainability, and climate change adaptability . Environ. Manage . 63 , 159–172. 10.1007/s00267-018-1117-3 [ PubMed ] [ CrossRef ] [ Google Scholar ]
- Ahmed N., Ward J. D., Saint C. P. (2014). Can integrated aquaculture-agriculture (IAA) produce “more crop per drop” . Food Sec . 6 , 767–779. 10.1007/s12571-014-0394-9 [ CrossRef ] [ Google Scholar ]
- Alexander K. S., Parry L., Thammavong P., Sacklokham S., Pasouvang S., Connell J. G., et al.. (2018). Rice farming systems in Southern Lao PDR: interpreting farmers' agricultural production decisions using Q methodology . Agric. Sys . 160 , 1–10. 10.1016/j.agsy.2017.10.018 [ CrossRef ] [ Google Scholar ]
- Ali S., Eum H. I., Cho J., Dan L., Khan F., Dairaku K., et al.. (2019). Assessment of climate extremes in future projections downscaled by multiple statistical downscaling methods over Pakistan . Atmos. Res . 222 , 114–133. 10.1016/j.atmosres.2019.02.009 [ CrossRef ] [ Google Scholar ]
- Allen C. D., Breshears D. D., McDowellm N. G. (2015). On underestimation of global vulnerability to tree mortality and forest die-off from hotter drought in the Anthropocene . Ecosphere . 6 , 1–55. 10.1890/ES15-00203.1 [ CrossRef ] [ Google Scholar ]
- Allen J., Pascual K. S., Romasanta R. R., Van Trinh M., Van Thach T., Van Hung N., et al.. (2020). Rice straw management effects on greenhouse gas emissions and mitigation options . Sustain. Rice Straw Manag. 9 , 145–159. 10.1007/978-3-030-32373-8_9 [ CrossRef ] [ Google Scholar ]
- Antle J. M. (2011). Parsimonious multi-dimensional impact assessment . Am. J. Agric. Econ . 93 , 1292–1311. 10.1093/ajae/aar052 [ CrossRef ] [ Google Scholar ]
- Antle J. M., Stoorvogel J. J., Valdivia R. O. (2014). New parsimonious simulation methods and tools to assess futurefood and environmental security of farm populations . Philos. Trans. Soc. Biol. Sci . 369 , 2012–2028. 10.1098/rstb.2012.0280 [ PMC free article ] [ PubMed ] [ CrossRef ] [ Google Scholar ]
- Arehart J. H., Hart J., Pomponi F., D'Amico B. (2021). Carbon sequestration and storage in the built environment . Sustain. Prod. Consum . 27 , 1047–1063. 10.1016/j.spc.2021.02.028 [ CrossRef ] [ Google Scholar ]
- Arunrat N., Pumijumnong N., Hatano R. (2018). Predicting local-scale impact of climate change on rice yield and soil organic carbon sequestration: a case study in Roi Et Province, Northeast Thailand . Agric. Sys . 164 , 58–70. 10.1016/j.agsy.2018.04.001 [ CrossRef ] [ Google Scholar ]
- Aryal J. P., Sapkota T. B., Khurana R., Khatri-Chhetri A., Jat M. L. (2019). Climate change and agriculture in South Asia: adaptation options in smallholder production systems . Environ. Develop. Sustain . 20 , 1–31. 10.1007/s10668-019-00414-4 [ CrossRef ] [ Google Scholar ]
- Asseng S., Ewert F., Martre P., Rotter R. P. (2014). Rising temperatures reduce global wheat production . Nat. Climate Change 5 , 143–147. 10.1038/nclimate2470 [ CrossRef ] [ Google Scholar ]
- Asseng S., Martre P., Maiorano A., Rotter R. P., O'leary G. J., Fitzgerald G. J., et al.. (2019). Climate change impact and adaptation for wheat protein . Glob. Change Biol . 64 , 155–173. 10.1111/gcb.14481 [ PubMed ] [ CrossRef ] [ Google Scholar ]
- Azad N., Behmanesh J., Rezaverdinejad V., Tayfeh R. H. (2018). Climate change impacts modeling on winter wheat yield under full and deficit irrigation in Myandoab-Iran . Arch. Agron. Soil Sci . 64 , 731–746. 10.1080/03650340.2017.1373187 [ CrossRef ] [ Google Scholar ]
- Bailey-Serres J., Parker J. E., Ainsworth E. A., Oldroyd G. E., Schroeder J. I. (2018). Genetic strategies for improving crop yields . Nature 575 , 109–118. 10.1038/s41586-019-1679-0 [ PMC free article ] [ PubMed ] [ CrossRef ] [ Google Scholar ]
- Balamurugan B., Tejaswi V., Priya K., Sasikala R., Karuthadurai T., Ramamoorthy M., et al.. (2018). Effect of global warming on livestock production and reproduction: an overview . Res. Rev . 6 , 12–18. [ Google Scholar ]
- Bao Y., Wang F., Tong S., Na L., Han A., Zhang J., et al.. (2019). Effect of drought on outbreaks of major forest pests, pine caterpillars ( Dendrolimus spp.), in Shandong Province, China . Forests 10 , 264–272. 10.3390/f10030264 [ CrossRef ] [ Google Scholar ]
- Bett B., Lindahl J., Delia G. (2019). Climate change and infectious livestock diseases: the case of Rift Valley fever and tick-borne diseases . Climate Smart Agri. Pap . 3 , 29–37. 10.1007/978-3-319-92798-5_3 [ CrossRef ] [ Google Scholar ]
- Bheemanahalli R., Vennam R. R., Ramamoorthy P., Reddy K. R. (2022). Effects of post-flowering heat and drought stresses on physiology, yield, and quality in maize ( Zea mays L.). Plant Stress 2022, 100106. 10.1016/j.stress.2022.100106 [ CrossRef ] [ Google Scholar ]
- Boonwichai S., Shrestha S., Babel M. S., Weesakul S., Datta A. (2019). Evaluation of climate change impacts and adaptation strategies on rainfed rice production in Songkhram River Basin, Thailand . Sci. Total Environ . 652 , 189–201. 10.1016/j.scitotenv.2018.10.201 [ PubMed ] [ CrossRef ] [ Google Scholar ]
- Cai Y., Bandara J. S., Newth D. A. (2016). Framework for integrated assessment of food production economics in South Asia under climate change . Environ. Model. Software 75 , 459–497. 10.1016/j.envsoft.2015.10.024 [ CrossRef ] [ Google Scholar ]
- Challinor A. J., Muller C., Asseng S., Deva C., Nicklin K. J., Wallach D., et al.. (2018). Improving the use of crop models for risk assessment and climate change adaptation . Agric. Sys . 159 , 296–306. 10.1016/j.agsy.2017.07.010 [ PMC free article ] [ PubMed ] [ CrossRef ] [ Google Scholar ]
- Chen H., Liang Z., Liu Y., Jiang Q., Xie S. (2018). Effects of drought and flood on crop production in China across 1949–2015: spatial heterogeneity analysis with Bayesian hierarchical modeling . Natural Hazards 92 , 525–541. 10.1007/s11069-018-3216-0 [ CrossRef ] [ Google Scholar ]
- Chen S., Yong Y., Ju X. (2021). Effect of heat stress on growth and production performance of livestock and poultry: mechanism to prevention . J. Thermal Biol . 99, 103019. 10.1016/j.jtherbio.2021.103019 [ PubMed ] [ CrossRef ] [ Google Scholar ]
- Chitale V., Silwal R., Matin M. (2018). Assessing the impacts of climate change on distribution of major non-timber forest plants in Chitwan Annapurna Landscape, Nepal . Resources 4 , 7–66. 10.3390/resources7040066 [ CrossRef ] [ Google Scholar ]
- Chow J., Khanom T., Hossain R., Khadim J. (2019). Forest management for climate change adaptation in Bangladesh . Confront. Climate Change Bangladesh 2 , 39–50. 10.1007/978-3-030-05237-9_4 [ CrossRef ] [ Google Scholar ]
- Chun J. A., Li S., Wang Q., Lee W. S., Lee E. J., Horstmann N., et al.. (2016). Assessing rice productivity and adaptation strategies for Southeast Asia under climate change through multi-scale crop modeling . Agric. Sys . 143 , 14–21. 10.1016/j.agsy.2015.12.001 [ CrossRef ] [ Google Scholar ]
- Clair S. B. S., Lynch J. P. (2010). The opening of Pandora's Box: climate change impacts on soil fertility and crop nutrition in developing countries . Plant Soil . 335 , 101–115. 10.1007/s11104-010-0328-z [ CrossRef ] [ Google Scholar ]
- Dagar J. C., Minhas P. S. (2016). Saline irrigation for productive agroforestry systems ,> in Agroforestry for the Management of Waterlogged Saline Soils and Poor-Quality Waters, Advances in Agroforestry , eds J. C. Dagar and P. S. Minhas (New Delhi: Springer), 13 , 145–161. 10.1007/978-81-322-2659-8_9 [ CrossRef ] [ Google Scholar ]
- Dagar J. C., Yadav R. K. (2017). Climate resilient approaches for enhancing productivity of saline agriculture . J. Soil Salinity Water Qual . 9 , 9–21. [ Google Scholar ]
- Das S. K. (2018). Impact of climate change (heat stress) on livestock: adaptation and mitigation strategies for sustainable production . Agric. Rev . 39 , 35–42. 10.18805/ag.R-1777 [ CrossRef ] [ Google Scholar ]
- De Zoysa M., Inoue M. (2014). Climate change impacts, agro-forestry adaptation and policy environment in Sri Lanka . Open J. Forest . 28 , 400–439. 10.4236/ojf.2014.45049 [ CrossRef ] [ Google Scholar ]
- Degani E., Leigh S. G., Barber H. M., Jones H. E., Lukac M., Sutton P., et al.. (2019). Crop rotations in a climate change scenario: short-term effects of crop diversity on resilience and ecosystem service provision under drought . Agric. Ecosys. Environ . 285, 106625. 10.1016/j.agee.2019.106625 [ CrossRef ] [ Google Scholar ]
- Din M. S. U., Mubeen M., Hussain S., Ahmad A., Hussain N., Ali M. A., et al.. (2022). World nations priorities on climate change and food security ,> in Building Climate Resilience in Agriculture (Cham: Springer; ), 365–384. 10.1007/978-3-030-79408-8_22 [ CrossRef ] [ Google Scholar ]
- Downing M. M. R., Nejadhashemi A. P., Harrigan T., Woznicki S. A. (2017). Climate change and livestock: impacts, adaptation, and mitigation . Climate Risk Manag . 16 , 145–163. 10.1016/j.crm.2017.02.001 [ CrossRef ] [ Google Scholar ]
- Dubey A., Malla M. A., Khan F., Chowdhary K., Yadav S., Kumar A., et al.. (2019). Soil microbiome: a key player for conservation of soil health under changing climate . Biodive. Conserv . 28 , 2405–2429. 10.1007/s10531-019-01760-5 [ CrossRef ] [ Google Scholar ]
- Ebi K. L., Loladze I. (2019). Elevated atmospheric CO 2 concentrations and climate change will affect our food's quality and quantity . Lancet Planetary Health 3 , 283–284. 10.1016/S2542-5196(19)30108-1 [ PubMed ] [ CrossRef ] [ Google Scholar ]
- Ewert F., Rötter R. P., Bindi M., Webber H., Trnka M., Kersebaum K. C., et al.. (2015). Crop modelling for integrated assessment of risk to food production from climate change . Environ. Model Soft . 26 , 287–303. 10.1016/j.envsoft.2014.12.003 [ CrossRef ] [ Google Scholar ]
- Fahad S., Bajwa A. A., Nazir U., Anjum S. A., Farooq A., Zohaib A., et al.. (2017). Crop production under drought and heat stress: plant responses and management options . Fron. Plant Sci . 9, 1147. 10.3389/fpls.2017.01147 [ PMC free article ] [ PubMed ] [ CrossRef ] [ Google Scholar ]
- FAO (2016). The Impact of Disasters on Agriculture: Addressing the Information Gap . Rome, 19. [ Google Scholar ]
- FAO IFAD, and WFP. (2015). The State of Food Insecurity in the World: Meeting the 2015 International Hunger Targets: Taking Stock of Uneven Progress. FAO, IFAD and WFP . Rome: FAO. [ PMC free article ] [ PubMed ] [ Google Scholar ]
- Fujimori S., Wu W., Doelman J., Frank S., Hristov J., Kyle P., et al.. (2021). Impacts of GHG Emissions Abatement Measures on Agricultural Market and Food Security (London: Nature Food; ). 10.21203/rs.3.rs-128167/v1 [ CrossRef ] [ Google Scholar ]
- Garcia-Franco N., Hobley E., Hübner R., Wiesmeier M. (2018). Climate-smart soil management in semiarid regions . Soil Manag. Climate Change 12 , 349–368. 10.1016/B978-0-12-812128-3.00023-9 [ CrossRef ] [ Google Scholar ]
- Garlock T., Asche F., Anderson J., Ceballos-Concha A., Love D. C., Osmundsen T. C., et al.. (2022). Aquaculture: the missing contributor in the food security agenda . Global Food Sec . 32, 100620. 10.1016/j.gfs.2022.100620 [ CrossRef ] [ Google Scholar ]
- Garnett T. (2011). Where are the best opportunities for reducing greenhouse gas emissions in the food system (including the food chain)? Food Policy 36 , S23–S32. 10.1016/j.foodpol.2010.10.010 [ CrossRef ] [ Google Scholar ]
- Geng X., Wang F., Ren W., Hao Z. (2019). Climate change impacts on winter wheat yield in Northern China . Adv. Meteorol . 4 , 11–12. 10.1155/2019/2767018 [ CrossRef ] [ Google Scholar ]
- Ghaffar A., Habib M. H. R., Ahmad S., Ahmad I., Khan M. A., Hussain J., et al.. (2022). Adaptations in Cropping System and Pattern for Sustainable Crops Production under Climate Change Scenarios . (Boca Raton, FL: CRC Press), 10. 10.1201/9781003286417-1 [ CrossRef ] [ Google Scholar ]
- Gouldson A., Colenbrander S., Sudmant A., Papargyropoulou E., Kerr N., McAnulla F., et al.. (2016). Cities and climate change mitigation: economic opportunities and governance challenges in Asia . Cities 54 , 11–19. 10.1016/j.cities.2015.10.010 [ CrossRef ] [ Google Scholar ]
- Greenwood S., Ruiz-Benito P., Martinez-Vilalta J., Lloret F., Kitzberger T., Allen C. D., et al.. (2017). Tree mortality across biomes is promoted by drought intensity, lower wood density and higher specific leaf area . Ecol. Lett . 20 , 539–553. 10.1111/ele.12748 [ PubMed ] [ CrossRef ] [ Google Scholar ]
- Guo H., Bao A., Ndayisaba F., Liu T., Jiapaer G., El-Tantawi A. M., et al.. (2018). Space-time characterization of drought events and their impacts on vegetation in Central Asia . J. Hydrol . 564 , 1165–1178. 10.1016/j.jhydrol.2018.07.081 [ CrossRef ] [ Google Scholar ]
- Habeeb A. A., Gad A. E., Atta A. M. (2018). Temperature-humidity indices as indicators to heat stress of climatic conditions with relation to production and reproduction of farm animals . Int. J. Biotechnol. Recent Adv . 1 , 35–50. 10.18689/ijbr-1000107 [ CrossRef ] [ Google Scholar ]
- Handisyde N., Telfer T. C., Ross L. G. (2017). Vulnerability of aquaculture-related livelihoods to changing climate at the global scale . Fish Fisheries 18 , 466–488. 10.1111/faf.12186 [ CrossRef ] [ Google Scholar ]
- Hanich Q., Wabnitz C. C., Ota Y., Amos M., Donato-Hunt C., Hunt A. (2018). Small-scale fisheries under climate change in the Pacific Islands region . Mar. Policy 88 , 279–284. 10.1016/j.marpol.2017.11.011 [ CrossRef ] [ Google Scholar ]
- Hanjra M. A., Qureshi M. E. (2010). Global water crisis and future food security in an era of climate change . Food Policy . 35 , 365–377. 10.1016/j.foodpol.2010.05.006 [ CrossRef ] [ Google Scholar ]
- Hashida Y., Lewis D. J. (2019). The intersection between climate adaptation, mitigation, and natural resources: an empirical analysis of forest management . J. Asso. Environ. Res. Econo . 18 , 6893–6926. 10.1086/704517 [ CrossRef ] [ Google Scholar ]
- Hasnat G. T., Kabir M. A., Hossain M. A. (2019). Major environmental issues and problems of South Asia, Particularly Bangladesh . Handb. Environ. Mater. Manag . 7 , 109–148. 10.1007/978-3-319-73645-7_7 [ CrossRef ] [ Google Scholar ]
- Hassen A., Talore D. G., Tesfamariam E. H., Friend M. A., Mpanza T. D. E. (2017). Potential use of forage-legume intercropping technologies to adapt to climate-change impacts on mixed crop-livestock systems in Africa: a review . Regional Environ. Change 68 , 1713–1724 10.1007/s10113-017-1131-7 [ CrossRef ] [ Google Scholar ]
- Havlik P., Valin H., Mosnier A., Obersteiner M., Baker J. S., Herrero M., et al.. (2013). Crop productivity and the global livestock sector: implications for land use change and greenhouse gas emissions . Am. J. Agric. Econ . 95 , 442–448. 10.1093/ajae/aas085 [ CrossRef ] [ Google Scholar ]
- Henderson B. B., Gerber P. J., Hilinski T. E., Falcucci A., Ojima D. S., Salvatore M., et al.. (2015). Greenhouse gas mitigation potential of the world's grazing lands: modeling soil carbon and nitrogen fluxes of mitigation practices . Agric. Ecosyst. Environ . 207 , 91–100. 10.1016/j.agee.2015.03.029 [ CrossRef ] [ Google Scholar ]
- Henry B., Charmley E., Eckard R., Gaughan J. B., Hegarty R. (2012). Livestock production in a changing climate: adaptation and mitigation research in Australia . Crop Pasture Sci . 63 , 191–202. 10.1071/CP11169 [ CrossRef ] [ Google Scholar ]
- Herrero M., Henderson B., Havlik P., Thornton P. K., Conant R. T., Smith P., et al.. (2016). Greenhouse gas mitigation potentials in the livestock sector . Nat. Climate Change 6 , 452–461. 10.1038/nclimate2925 [ CrossRef ] [ Google Scholar ]
- Hilmi N., Osborn D., Acar S., Bambridge T., Chlous F., Cinar M., et al.. (2019). Socio-economic tools to mitigate the impacts of ocean acidification on economies and communities reliant on coral reefs–a framework for prioritization . Reg. Stud. Mar. Sci . 100 , 559–566. 10.1016/j.rsma.2019.100559 [ CrossRef ] [ Google Scholar ]
- Hisano M., Searle E. B., Chen H. Y. (2018). Biodiversity as a solution to mitigate climate change impacts on the functioning of forest ecosystems . Biol. Rev . 93 , 439–456. 10.1111/brv.12351 [ PubMed ] [ CrossRef ] [ Google Scholar ]
- Hoogenboom G., Jones J. W., Wilkens P. W., Porter C. H., Boote K. J., Hunt L. A., et al.. (2011). Decision Support System for Agro-Technology System Transfer, Version 4.5 (CD-ROM). Honolulu: University of Hawaii, 69–77. [ Google Scholar ]
- Hoogenboom G., Jones J. W., Wilkens P. W., Porter C. H., Boote K. J., Hunt L. A., et al.. (2015). Decision Support System for Agrotechnology Transfer (DSSAT). Version 4.6 . Prosser, WA: DSSAT Foundation. [ Google Scholar ]
- Hoogenboom G., Porter C. H., Shelia V., Boote K. J., Singh U., White J. W., et al.. (2019). Decision Support System for Agrotechnology Transfer (DSSAT) Version 4.7.5.0 ( www.DSSAT.net ) . Gainesville, FL: DSSAT Foundation. [ Google Scholar ]
- Hussain J., Khaliq T., Ahmad A., Akhter J., Asseng S. (2018). Wheat responses to climate change and its adaptations: a focus on arid and semi-arid environment . Int. J. Environ. Res . 12 , 117–126. 10.1007/s41742-018-0074-2 [ CrossRef ] [ Google Scholar ]
- Hussein H. A. A., Alshammari S. O., Kenawy S. K., Elkady F. M., Badawy A. A. (2022). Grain-priming with L-arginine improves the growth performance of wheat ( Triticum aestivum L.) plants under drought stress . Plants 11 , 1219. 10.3390/plants11091219 [ PMC free article ] [ PubMed ] [ CrossRef ] [ Google Scholar ]
- Iannella M., De Simone W., D'Alessandro P., Biondi M. (2021). Climate change favours connectivity between virus-bearing pest and rice cultivations in sub-Saharan Africa, depressing local economies . PeerJ . 9, e12387. 10.7717/peerj.12387 [ PMC free article ] [ PubMed ] [ CrossRef ] [ Google Scholar ]
- Imam N., Hossain M. K., Saha T. R. (2017). Potentials and challenges of using ICT for climate change adaptation: a study of vulnerable community in riverine islands of bangladesh ,> in Catalyzing Development Through ICT Adoption , eds H. Kaur, E. Lechman, and A. Marszk (Cham: Springer; ), 89–110. 10.1007/978-3-319-56523-1_7 [ CrossRef ] [ Google Scholar ]
- IPCC (2019). Global warming of 1.5 ° C. Summary for Policy Makers. Switzerland: World Meteorological Organization, United Nations Environment Program, and Intergovernmental Panel on Climate Change . Bern. [ Google Scholar ]
- Iqbal N., Sehar Z., Fatma M., Umar S., Sofo A., Khan N. A. (2022). Nitric oxide and abscisic acid mediate heat stress tolerance through regulation of osmolytes and antioxidants to protect photosynthesis and growth in wheat plants . Antioxidants 11 , 372. 10.3390/antiox11020372 [ PMC free article ] [ PubMed ] [ CrossRef ] [ Google Scholar ]
- Islam M. S., Haq M. E. (2018). Vulnerability of aquaculture-based fish production systems to the impacts of climate change: insights from Inland Waters in Bangladesh . Bangladesh I 6 , 67–97. 10.1007/978-3-319-26357-1_3 [ CrossRef ] [ Google Scholar ]
- Jahan I., Ahsan D., Farque M. H. (2017). Fishers' local knowledge on impact of climate change and anthropogenic interferences on Hilsa fishery in South Asia: evidence from Bangladesh . Environ. Develop. Sustain . 17 , 461–478. 10.1007/s10668-015-9740-0 [ CrossRef ] [ Google Scholar ]
- Jhariya M. K., Yadav D. K., Banerjee A., Raj A., Meena R. S. (2019). Sustainable forestry under changing climate . Sustain. Agric. Forest. Environ Manag . 24 , 285–326. 10.1007/978-981-13-6830-1_9 [ CrossRef ] [ Google Scholar ]
- Kalas P. P. (2009). Planting the Knowledge Seed Adapting to climate change using ICTs Concepts, Current Knowledge and Innovative Examples . Pretoria: Building Communication Opportunities (BCO) Alliance. 10.13140/RG.2.2.17959.85921 [ CrossRef ] [ Google Scholar ]
- Karanasios S. T. A. N. (2011). New and Emergent ICTs and Climate Change in Developing Countries . Manchester: Center for Development Informatics, Institute for Development Policy and Management, SED, University of Manchester. [ Google Scholar ]
- Karmakar S., Purkait S., Das A., Samanta R., Kumar K. (2018). Climate change and Inland fisheries: impact and mitigation strategies . J. Exp. Zool. Ind . 21, 329–335. Avaiable online at: https://www.researchgate.net/publication/323546601_CLIMATE_CHANGE_AND_INLAND_FISHERIES_IMPACT_AND_MITIGATION_STRATEGIES
- Kataria S., Verma S. K. (2018). Salinity stress responses and adaptive mechanisms in major glycophytic crops: the story so far . Salinity Responses Tolerance Plants 1 , 1–39. 10.1007/978-3-319-75671-4_1 [ CrossRef ] [ Google Scholar ]
- Keating B. A., Carberry P. S., Hammer G. L., Probert M. E. (2003). An overview of APSIM a model designed for farming systems simulation . Eur. J. Agron . 18 , 267–288. 10.1016/S1161-0301(02)00108-9 [ CrossRef ] [ Google Scholar ]
- Keenan R. J. (2015). Climate change impacts and adaptation in forest management: a review . Ann. For. Sci . 72 , 145–167. 10.1007/s13595-014-0446-5 [ CrossRef ] [ Google Scholar ]
- Kheir A. M., El Baroudy A., Aiad M. A., Zoghdan M. G., El-Aziz M. A. A., Ali M. G., et al.. (2019). Impacts of rising temperature, carbon dioxide concentration and sea level on wheat production in North Nile delta . Sci. Total Environ . 651 , 3161–3173. 10.1016/j.scitotenv.2018.10.209 [ PubMed ] [ CrossRef ] [ Google Scholar ]
- Kolstrom M., Lindner M., Vilen T., Maroschek M., Seidl R., Lexer M. J., et al.. (2011). Reviewing the science and implementation of climate change adaptation measures in European forestry . Forest 2 , 961–982. 10.3390/f2040961 [ CrossRef ] [ Google Scholar ]
- Kumar S., Dwivedi S. K., Basu S., Kumar G., Mishra J. S., Koley T. K., et al.. (2020). Anatomical, agro-morphological and physiological changes in rice under cumulative and stage specific drought conditions prevailed in eastern region of India . Field Crops Res . 245, 107658. 10.1016/j.fcr.2019.107658 [ CrossRef ] [ Google Scholar ]
- Kumar V. S., Kumar R. P., Harikrishna C. H., Rani M. S. (2018). Effect of heat stress on production and reproduction performance of buffaloes-a review . Pharma Innov . 7, 629–633. Available online at: https://www.thepharmajournal.com/archives/2018/vol7issue4/PartJ/7-3-162-175.pdf
- Kurukulasuriya P., Rosenthal S. (2003). Climate Change and Agriculture: A Review of Impacts and Adaptations. Climate Change Series Paper No. 91 . Washington, DC: World Bank. [ Google Scholar ]
- Lal R. (2007). Carbon management in agricultural soils . Mitig. Adapt. Strateg. Glob. Chang . 12 , 303–322. 10.1007/s11027-006-9036-7 [ CrossRef ] [ Google Scholar ]
- Lam V. W., Chavanich S., Djoundourian S., Dupont S., Gaill F., Holzer G., et al.. (2019). Dealing with the effects of ocean acidification on coral reefs in the Indian Ocean and Asia . Reg. Stud. Marine Sci . 100 , 560–570. 10.1016/j.rsma.2019.100560 [ CrossRef ] [ Google Scholar ]
- Liang C., Xian W., Pauly D. (2018). Impacts of ocean warming on China's fisheries catches: an application of “mean temperature of the catch” concept . Front. Mar. Sci . 6 , 5–26. 10.3389/fmars.2018.00026 [ CrossRef ] [ Google Scholar ]
- Lima V. P., de Lima R. A. F., Joner F., Siddique I., Raes N., Ter Steege H. (2022). Climate change threatens native potential agroforestry plant species in Brazil . Sci. Rep . 12 , 1–14. 10.1038/s41598-022-06234-3 [ PMC free article ] [ PubMed ] [ CrossRef ] [ Google Scholar ]
- Liu J., Hasanuzzaman M., Wen H., Zhang J., Peng T., Sun H., et al.. (2019). High temperature and drought stress cause abscisic acid and reactive oxygen species accumulation and suppress seed germination growth in rice . Protoplasma 256 , 1217–1227. 10.1007/s00709-019-01354-6 [ PubMed ] [ CrossRef ] [ Google Scholar ]
- Liu Y., Chen Q., Ge Q., Dai J., Qin Y., Dai L., et al.. (2018). Modelling the impacts of climate change and crop management on phenological trends of spring and winter wheat in China . Agric. For. Meteorol . 248 , 518–526. 10.1016/j.agrformet.2017.09.008 [ CrossRef ] [ Google Scholar ]
- Lv Z., Liu X., Cao W., Zhu Y. (2013). Climate change impacts on regional winter wheat production in main wheat production regions of China . Agric. For. Meteorol . 171 , 234–248. 10.1016/j.agrformet.2012.12.008 [ CrossRef ] [ Google Scholar ]
- Lybbert T. J., Sumner D. A. (2012). Agricultural technologies for climate change in developing countries: policy options for innovation and technology diffusion . Food Policy 37 , 114–123. 10.1016/j.foodpol.2011.11.001 [ CrossRef ] [ Google Scholar ]
- Ma Q., Zhang J., Sun C., Zhang F., Wu R., Wu L. (2018). Drought characteristics and prediction during pasture growing season in Xilingol grassland, northern China . Theo. App. Climatol . 133 , 165–178. 10.1007/s00704-017-2150-5 [ CrossRef ] [ Google Scholar ]
- Mbow C., Smith P., Skole D., Duguma L., Bustamante M. (2014). Achieving mitigation and adaptation to climate change through sustainable agroforestry practices in Africa . Curr. Opin. Environ. Sustain . 6 , 8–14. 10.1016/j.cosust.2013.09.002 [ CrossRef ] [ Google Scholar ]
- Meena R. K., Verma T. P., Yadav R. P., Mahapatra S. K., Surya J. N., Singh D., et al.. (2019). Local perceptions and adaptation of indigenous communities to climate change: Evidences from High Mountain Pangi valley of Indian Himalayas . Ind. J. Trad. Knowl. 11 , 876–888. Available online at: https://www.researchgate.net/publication/343818597_Local_perceptions_and_adaptation_of_indigenous_communities_to_climate_change_Evidences_from_High_Mountain_Pangi_valley_of_Indian_Himalaya [ Google Scholar ]
- Meera S. N., Balaji V., Muthuraman P., Sailaja B., Dixit S. (2012). Changing roles of agricultural extension: harnessing information and communication technology (ICT) for adapting to stresses envisaged under climate change . Crop Stress Manag . 16 , 585–605. 10.1007/978-94-007-2220-0_19 [ CrossRef ] [ Google Scholar ]
- Moreira S. L., Pires C. V., Marcatti G. E., Santos R. H., Imbuzeiro H. M., Fernandes R. B. (2018). Intercropping of coffee with the palm tree, macauba, can mitigate climate change effects . Agric. Forest. Meteorol . 265 , 379–390. 10.1016/j.agrformet.2018.03.026 [ CrossRef ] [ Google Scholar ]
- Mottaleb K. A., Rejesus R. M., Murty M. V. R., Mohanty S., Li T. (2017). Benefits of the development and dissemination of climate-smart rice: ex ante impact assessment of drought-tolerant rice in South Asia . Miti. Adap. Strat. Global Change 22 , 879–901. 10.1007/s11027-016-9705-0 [ CrossRef ] [ Google Scholar ]
- Myers S. S., Smith M. R., Guth S., Golden C. D., Vaitla B., Mueller N. D., et al.. (2017). Climate change and global food systems: potential impacts on food security and undernutrition . Ann. Rev. Public Health . 38 , 259–277. 10.1146/annurev-publhealth-031816-044356 [ PubMed ] [ CrossRef ] [ Google Scholar ]
- Nasi W., Amin A., Fahad S. (2018). Future risk assessment byestimating historical heat wave trends with projected heat accumulation using SimCLIM climate model in Pakistan . Atmos. Res . 205 , 118–133. 10.1016/j.atmosres.2018.01.009 [ CrossRef ] [ Google Scholar ]
- Nasir I. R., Rasul F., Ahmad A. (2020). Climate change impacts and adaptations for fine, coarse, and hybrid rice using CERES-Rice . Environ. Sci. Pollut. Res . 27 , 9454–9464. 10.1007/s11356-019-07080-z [ PubMed ] [ CrossRef ] [ Google Scholar ]
- Nguyen H., Morrison J., Neven D. (2019). Changing food systems: implications for food security and nutrition . Sustain Food Agric . 21 , 153–168. 10.1016/B978-0-12-812134-4.00009-1 [ CrossRef ] [ Google Scholar ]
- Nguyen N. H. (2015). Genetic improvement for important farmed aquaculture species with a reference to carp, tilapia and prawns in Asia: achievements, lessons and challenges . Fish Fisheries 17 :483–506 10.1111/faf.12122 [ CrossRef ] [ Google Scholar ]
- Nizam H. A., Zaman K., Khan K. B., Batool R., Khurshid M. A., Shoukry A. M., et al.. (2020). Achieving environmental sustainability through information technology:“Digital Pakistan” initiative for green development . Environ. Sci. Poll. Res . 2 , 1–16. 10.1007/s11356-020-07683-x [ PubMed ] [ CrossRef ] [ Google Scholar ]
- Noori M. S., Taliman N. A. (2022). Effect of drought stress and chemical fertilizers on wheat productivity and grain and quality . Plant Prod. Gene 3 , 43–56. 10.34785/J020.2022.800 [ CrossRef ] [ Google Scholar ]
- Obermeier W. A., Lehnert L. W., Kammann C. I., Muller C., Grunhage L., Luterbacher J., et al.. (2017). Reduced CO 2 fertilization effect in temperate C 3 grasslands under more extreme weather conditions . Nat. Climate Change 7 , 137–148. 10.1038/nclimate3191 [ CrossRef ] [ Google Scholar ]
- Ogello E. O., Mlingi F. T., Nyonje B. M., Charo-Karisa H., Munguti J. M. (2013). Can integrated livestock-fish culture be a solution to East Afircan's food insecurity a review . Afr. J. Food Agric. Nut. Develop . 13 , 8058–8078. 10.18697/ajfand.59.12920 [ CrossRef ] [ Google Scholar ]
- Ottaviani D., De Young C., Tsuji S. (2017). Assessing water availability and economic social and nutritional contributions from inland capture fisheries and aquaculture: an indicator-based framework: a compilation of water-related indicators in selected African and Asian Countries . FAO Fisheries Aquacult. Circul . 11 , 16–21. [ Google Scholar ]
- Paricha A. M. P., Sethi K. C., Gupta V., Pathak A., Chhotray S. K. (2017). Soil water conservation for microcatchment water harvesting systems . J. Soil Sci. Plant Nutri . 8 , 55–59. [ Google Scholar ]
- Phand S., Pankaj P. K. (2021). Climate-resilient livestock farming to ensure food and nutritional security ,> in Climate Change and Resilient Food Systems (Singapore: Springer; ), 381–398. 10.1007/978-981-33-4538-6_15 [ CrossRef ] [ Google Scholar ]
- Pineiro G., Paruelo J. M., Oesterheld M., Jobbagy E. G. (2010). Pathways of grazing effects on soil organic carbon and nitrogen . Range Ecol. Manag . 63 , 109–119. 10.2111/08-255.1 [ CrossRef ] [ Google Scholar ]
- Poonam A., Saha S., Nayak P. K., Sinhababu D. P., Sahu P. K., Satapathy B. S., et al.. (2019). Rice-fish integrated farming systems for eastern India . J. Integrat. Agric . 6, 77–86. Available online at: https://www.semanticscholar.org/paper/Rice-Fish-Integrated-Farming-Systems-for-Eastern-Poonam-Saha/43040f2fe84dab3be27fd48785f040351278f25d
- Population of Asia (2019). Demographics: Density Ratios, Growth Rate, Clock, Rate of Men to Woman . Available online at: www. population of.net (accessed June 2, 2019). [ Google Scholar ]
- Prein M. (2002). Integration of aquaculture into crop–animal systems in Asia . Agric. Syst . 71 , 127–146. 10.1016/S0308-521X(01)00040-3 [ CrossRef ] [ Google Scholar ]
- Quyen N. H., Duong T. H., Yen B. T., Sebastian L. (2018). Impact of climate change on future rice production in the Mekong River Delta. Miti. Adap. Strat. Global Change .12, 55–59 . Available online at: https://hdl.handle.net/10568/99563
- Rahman M. H. U., Ahmad A., Wajid A. (2019). Application of CSM-CROPGRO-cotton model for cultivars and optimum planting dates: evaluation in changing semi-arid climate . Field Crop Res . 238 , 139–152. 10.1016/j.fcr.2017.07.007 [ CrossRef ] [ Google Scholar ]
- Rahman M. H. U., Ahmad A., Wang X., Wajid A., Nasim W., Hussain M., et al.. (2018). Multi-model projections of future climate and climate change impacts uncertainty assessment for cotton production in Pakistan . Agric. Forest Meteorol . 253 , 94–113. 10.1016/j.agrformet.2018.02.008 [ CrossRef ] [ Google Scholar ]
- Rakszegi M., Darko E., Lovegrove A., Molnár I., Lang L., Bed,o Z., et al.. (2019). Drought stress affects the protein and dietary fiber content of wholemeal wheat flour in wheat/Aegilops addition lines . PLoS ONE 14 , e0211892. 10.1371/journal.pone.0211892 [ PMC free article ] [ PubMed ] [ CrossRef ] [ Google Scholar ]
- Raman A., Verulkar S. B., Mandal N. P., Variar M., Shukla V. D., Dwivedi J. L., et al.. (2012). Drought yield index to select high yielding rice lines under different drought stress severities . Rice 5 , 1–12. 10.1186/1939-8433-5-31 [ PMC free article ] [ PubMed ] [ CrossRef ] [ Google Scholar ]
- Raman H., Uppal R. K., Raman R. (2019). Genetic solutions to improve resilience of canola to climate change . Genomic Designing Climate-Smart Oilseed Crops 8 , 75–131. 10.1007/978-3-319-93536-2_2 [ CrossRef ] [ Google Scholar ]
- Rana E., Thwaites R., Luck G. (2017). Trade-offs and synergies between carbon, forest diversity and forest products in Nepal community forests . Environ. Conserv . 44 , 5–13. 10.1017/S0376892916000448 [ CrossRef ] [ Google Scholar ]
- Rao N., Lawson E. T., Raditloaneng W. N., Solomon D., Angula M. N. (2019). Gendered vulnerabilities to climate change: insights from the semi-arid regions of Africa and Asia . Climate Develop . 11 , 14–26. 10.1080/17565529.2017.1372266 [ CrossRef ] [ Google Scholar ]
- Rasul G., Neupane N., Hussain A., Pasakhala B. (2019). Beyond hydropower: towards an integrated solution for water, energy and food security in South Asia . Int. J. Water Resour. Develop . 17 , 1–25. 10.1080/07900627.2019.1579705 [ CrossRef ] [ Google Scholar ]
- Raza M. M., Khan M. A., Arshad M., Sagheer M., Sattar Z., Shafi J., et al.. (2015). Impact of global warming on insects . Arch. Phytopathol. Plant Protection . 48 , 84–94. 10.1080/03235408.2014.882132 [ CrossRef ] [ Google Scholar ]
- Ren S., Qin Q., Ren H. (2019). Contrasting wheat phenological responses to climate change in global scale . Sci. Total Environ . 665 , 620–631. 10.1016/j.scitotenv.2019.01.394 [ PubMed ] [ CrossRef ] [ Google Scholar ]
- Renaudeau D., Collin A., Yahav S., De Basilio V., Gourdine J. L., Collier R. J. (2012). Adaptation to hot climate and strategies to alleviate heat stress in livestock production . Animal 6 , 707–728. 10.1017/S1751731111002448 [ PubMed ] [ CrossRef ] [ Google Scholar ]
- Rezaei E. E., Siebert S., Huging H., Ewert F. (2018). Climate change effect on wheat phenology depends on cultivar change . Sci. Rep . 8 , 48–91. 10.1038/s41598-018-23101-2 [ PMC free article ] [ PubMed ] [ CrossRef ] [ Google Scholar ]
- Rosenzweig C., Jones J. W., Hatfield J. L., Ruane A. C., Boote K. J. (2013). The agricultural model inter comparison and improvement project (AgMIP): protocols and pilot studies . Agric. For. Meteorol . 170 , 166–182. 10.1016/j.agrformet.2012.09.011 [ CrossRef ] [ Google Scholar ]
- Ruane A. C., Cecil L. D., Horton R. M., Gordon R., McCollum R., Brown D., et al.. (2013). Climate change impact uncertainties for maize in Panama: farm information, climate projections, and yield sensitivities . Agric. For. Meteorol . 170 , 132–145. 10.1016/j.agrformet.2011.10.015 [ CrossRef ] [ Google Scholar ]
- Ruane A. C., Goldberg R., Chryssanthacopoulos J. (2015). Climate forcing datasets for agricultural modeling: merged products for gap-filling and historical climate series estimation . Agric. Meteorol . 200 , 233–248. 10.1016/j.agrformet.2014.09.016 [ CrossRef ] [ Google Scholar ]
- Runkle B. R., SuvocCarev K., Reba M. L., Reavis C. W., Smith S. F., Chiu Y. L., et al.. (2018). Methane emission reductions from the alternate wetting and drying of rice fields detected using the eddy covariance method . Environ. Sci. Technol . 53 , 671–681. 10.1021/acs.est.8b05535 [ PubMed ] [ CrossRef ] [ Google Scholar ]
- Sangareswari M., Balasubramanian A., Palanikumaran B., Aswini D. (2018). Carbon sequestration potential of a few selected tree species in Coimbatore District, Tamil Nadu . Adv. Res . 2 , 1–7. 10.9734/AIR/2018/39676 [ CrossRef ] [ Google Scholar ]
- Sanz-Cobena A., Lassaletta L., Aguilera E., Del Prado A., Garnier J., Billen G., et al.. (2019). Strategies for greenhouse gas emissions mitigation in Mediterranean agriculture: a review . Agric. Ecosys. Environ . 238 , 5–24. 10.1016/j.agee.2016.09.038 [ CrossRef ] [ Google Scholar ]
- Sarkar U. K., Borah B. C. (2018). Flood plain wetland fisheries of India: with special reference to impact of climate change . Wetlands Ecol. Manag . 26 , 1–15. 10.1007/s11273-017-9559-6 [ CrossRef ] [ Google Scholar ]
- Scherr S. J., Shames S., Friedman R. (2012). From climate-smart agriculture to climate-smart landscapes . Agric. Food Sec . 1 , 12–17. 10.1186/2048-7010-1-12 [ CrossRef ] [ Google Scholar ]
- Seddon N., Turner B., Berry P., Chausson A., Girardin C. A. (2018). Why Nature-Based Solutions to Climate Change Must Be Grounded in Sound Biodiversity Science (England: Nature Climate Change; ), 65. 10.20944/preprints201812.0077.v1 [ CrossRef ] [ Google Scholar ]
- Shafiq F., Nadeem A., Ahsan K., Siddiq M. (2014). Role of ICT in climate change monitoring: a review study of ICT based climate change Monitoring services . Res. J. Recent Sci . 3 , 123–130. 10.4236/ajcc.2018.72010 [ CrossRef ] [ Google Scholar ]
- Shah L., Ali A., Yahya M., Zhu Y., Wang S., Si H., et al.. (2018). Integrated control of fusarium head blight and deoxynivalenol mycotoxin in wheat . Plant Pathol . 67 , 532–548. 10.1111/ppa.12785 [ CrossRef ] [ Google Scholar ]
- Shi W., Yin X., Struik P. C., Solis C., Xie F., Schmidt R. C., et al.. (2017). High day-and night-time temperatures affect grain growth dynamics in contrasting rice genotypes . J. Exper. Bot . 68 , 5233–5245. 10.1093/jxb/erx344 [ PMC free article ] [ PubMed ] [ CrossRef ] [ Google Scholar ]
- Smith P., Olesen J. E. (2010). Synergies between the mitigation of, and adaptation to, climate change in agriculture . J. Agric. Sci . 148 , 543–552. 10.1017/S0021859610000341 [ CrossRef ] [ Google Scholar ]
- Sohag A. A. M., Tahjib-Ul-Arif M., Brestic M., Afrin S., Sakil M. A., Hossain M. T., et al.. (2020). Exogenous salicylic acid and hydrogen peroxide attenuate drought stress in rice . Plant, Soil Environ . 66 , 7–13. 10.17221/472/2019-PSE [ CrossRef ] [ Google Scholar ]
- Southgate P. C., Lucas J. S. (2019). Principles of aquaculture . Aquaculture 3 , 19–40. 10.1007/s10499-012-9530-8 [ CrossRef ] [ Google Scholar ]
- Spittlehouse D. L. (2005). Integrating climate change adaptation into forest management . Forestry Chron . 81 , 691–695. 10.5558/tfc81691-5 [ CrossRef ] [ Google Scholar ]
- Suryadi F. X. (2020). Soil and Water Management Strategies for Tidal Lowlands in Indonesia . Boca Raton, FL: CRC Press. 10.1201/9780429333231 [ CrossRef ] [ Google Scholar ]
- Tan B. T., Fam P. S., Firdaus R. R., Tan M. L., Gunaratne M. S. (2021). Impact of climate change on rice yield in Malaysia: a panel data analysis . Agric . 11, 569. 10.3390/agriculture11060569 [ CrossRef ] [ Google Scholar ]
- Tariq M., Ahmad S., Fahad S. (2018). The impact of climate warmingand crop management on phenology of sunflower-based cropping systems in Punjab, Pakistan . Agric. For. Meteorol . 256 , 270–282. 10.1016/j.agrformet.2018.03.015 [ CrossRef ] [ Google Scholar ]
- Thornton P. K., Herrero M. (2010). Potential for reduced methane and carbon dioxide emissions from livestock and pasture management in the tropics . Proc. Nat. Acad. Sci. U. S. A . 107 , 19667–19672. 10.1073/pnas.0912890107 [ PMC free article ] [ PubMed ] [ CrossRef ] [ Google Scholar ]
- Tian B., Yu Z., Pei Y., Zhang Z., Siemann E., Wan S., et al.. (2019). Elevated temperature reduces wheat grain yield by increasing pests and decreasing soil mutualists . Pest Manag. Sci . 75 , 466–475. 10.1002/ps.5140 [ PubMed ] [ CrossRef ] [ Google Scholar ]
- Tonnang H. E., Sokame B. M., Abdel-Rahman E. M., Dubois T. (2022). Measuring and modelling crop yield losses due to invasive insect pests under climate change . Curr. Opin. Insect Sci . 2022, 100873. 10.1016/j.cois.2022.100873 [ PubMed ] [ CrossRef ] [ Google Scholar ]
- Ullah A., Ahmad I., Ahmad A. (2019). Assessing climate change impacts on pearl millet under arid and semi-arid environments usingCSM-CERES-Millet model . Environ. Sci. Pollut. Res . 26 , 6745–6757. 10.1007/s11356-018-3925-7 [ PubMed ] [ CrossRef ] [ Google Scholar ]
- UNO (2015). World Population Prospective. The 2015 Revision, Volume II: Demographic Profiles . New York, NY: United Nations Department of Economic and Social Affairs, 21–27 . Available online at: https://www.un.org/development/desa/pd/content/world-population-prospects-2015-revision-volume-ii-demographic-profiles
- van Wettere W. H., Kind K. L., Gatford K. L., Swinbourne A. M., Leu S. T., Hayman P. T., et al.. (2021). Review of the impact of heat stress on reproductive performance of sheep . J. Animal Sci. Biotechnol . 12 , 1–18. 10.1186/s40104-020-00537-z [ PMC free article ] [ PubMed ] [ CrossRef ] [ Google Scholar ]
- Wang X., Pederson N., Chen Z., Lawton K., Zhu C., Han S. (2019a). Recent rising temperatures drive younger and southern Korean pine growth decline . Sci. Total Environ . 649 , 1105–1116. 10.1016/j.scitotenv.2018.08.393 [ PubMed ] [ CrossRef ] [ Google Scholar ]
- Wang Y., Wang L., Zhou J., Hu S., Chen H., Xiang J., et al.. (2019b). Research progress on heat stress of rice at flowering stage . Rice Sci . 26 , 1–10. 10.1016/j.rsci.2018.06.009 [ CrossRef ] [ Google Scholar ]
- Wasaya A., Yasir T. A., Sarwar N., Mubeen K., Rajendran K., Hadifa A., et al.. (2022). Climate change and global rice security ,> in Modern Techniques of Rice Crop Production (Singapore: Springer; ), 13–26. 10.1007/978-981-16-4955-4_2 [ CrossRef ] [ Google Scholar ]
- Wassie M., Zhang W., Zhang Q., Ji K., Chen L. (2019). Effect of heat stress on growth and physiological traits of alfalfa ( Medicago sativa L.) and a comprehensive evaluation for heat tolerance . Agron 9 , 597. 10.3390/agronomy9100597 [ CrossRef ] [ Google Scholar ]
- Williams P. A., Crespo O., Abu M. (2019). Adapting to changing climate through improving adaptive capacity at the local level–the case of smallholder horticultural producers in Ghana . Climate Risk Manag . 23 ,124–135. 10.1016/j.crm.2018.12.004 [ CrossRef ] [ Google Scholar ]
- World Bank (2018). World BankOpen Data. Available online at: https://data.worldbank.org/.com (accessed January, 2021).
- Xiong D. P., Shi P. L., Zhang X. Z., Zou C. B. (2016). Effects of grazing exclusion on carbon sequestration and plant diversity in grasslands of China: a meta-analysis . Ecol. Eng . 94 , 647–655. 10.1016/j.ecoleng.2016.06.124 [ CrossRef ] [ Google Scholar ]
- Xu J., Henry A., Sreenivasulu N. (2020). Rice yield formation under high day and night temperatures-a prerequisite to ensure future food security . Plant Cell Environ . 43 , 1595–1608. 10.1111/pce.13748 [ PubMed ] [ CrossRef ] [ Google Scholar ]
- Xu P., Zhou T., Zhao X., Luo H., Gao S., Li Z., et al.. (2018). Diverse responses of different structured forest to drought in Southwest China through remotely sensed data . Int. J. App. Earth Observ. Geoinform . 69 , 217–225. 10.1016/j.jag.2018.03.009 [ CrossRef ] [ Google Scholar ]
- Xu Y., Chu C., Yao S. (2021). The impact of high-temperature stress on rice: challenges and solutions . Crop J . 9 , 963–976. 10.1016/j.cj.2021.02.011 [ CrossRef ] [ Google Scholar ]
- Yadav S. S., Lal R. (2018). Vulnerability of women to climate change in arid and semi-arid regions: the case of India and South Asia . J. Arid Environ . 149 , 4–17. 10.1016/j.jaridenv.2017.08.001 [ CrossRef ] [ Google Scholar ]
- Yang H., Gu X., Ding M., Lu W., Lu D. (2018). Heat stress during grain filling affects activities of enzymes involved in grain protein and starch synthesis in waxy maize . Sci. Rep . 8 , 1–9. 10.1038/s41598-018-33644-z [ PMC free article ] [ PubMed ] [ CrossRef ] [ Google Scholar ]
- Yang Z., Zhang Z., Zhang T., Fahad S., Cui K., Nie L., et al.. (2017). The effect of season-long temperature increases on rice cultivars grown in the central and southern regions of China . Front. Plant Sci . 8 , 1908–1927. 10.3389/fpls.2017.01908 [ PMC free article ] [ PubMed ] [ CrossRef ] [ Google Scholar ]
- Yu H., Zhang Q., Sun P., Song C. (2018). Impact of droughts on winter wheat yield in different growth stages during 2001–2016 in Eastern China . Int. J. Disaster Risk Sci . 9 , 376–391. 10.1007/s13753-018-0187-4 [ CrossRef ] [ Google Scholar ]
- Zafar S. A., Hameed A., Nawaz M. A., Wei M. A., Noor M. A., Hussain M. (2018). Mechanisms and molecular approaches for heat tolerance in rice ( Oryza sativa L.) under climate change scenario . J. Integrative Agric . 17 , 726–738. 10.1016/S2095-3119(17)61718-0 [ CrossRef ] [ Google Scholar ]
- Zahra N., Wahid A., Hafeez M. B., Ullah A., Siddique K. H. M., Farooq M. (2021). Grain development in wheat under combined heat and drought stress: Plant responses and management . Environ. Experi. Bot . 188, 104517. 10.1016/j.envexpbot.2021.104517 [ CrossRef ] [ Google Scholar ]
- Zanamwe N., Okunoye A. (2013). Role of information and communication technologies (ICTs) in mitigating, adapting to and monitoring climate change in developing countries . Int. Conf. ICT Africa, Harare, Zimbabwe 4 , 20–23. Available online at: https://www.semanticscholar.org/paper/Role-of-information-and-communication-technologies-Okunoye/4c987ba4dbef48157a2d287ecf2629ce242566e6 [ Google Scholar ]
- Zhao K., Tao Y., Liu M., Yang D., Zhu M., Ding J., et al.. (2022). Does temporary heat stress or low temperature stress similarly affect yield, starch, and protein of winter wheat grain during grain filling? J. Cereal Sci . 103, 103408. 10.1016/j.jcs.2021.103408 [ CrossRef ] [ Google Scholar ]
- Zhu X., Zhang S., Liu T., Liu Y. (2021). Impacts of heat and drought on gross primary productivity in China . Remote Sens . 13, 378. 10.3390/rs13030378 [ CrossRef ] [ Google Scholar ]
10+ SAMPLE Agricultural Project Proposal in PDF
Agricultural project proposal, 10+ sample agricultural project proposal, what is an agricultural project proposal, what is agriculture, types of agricultural products, benefits of agricultural projects, tips on how to write an agricultural project proposal, what are some examples of agricultural project proposal objectives, what are some different types of agriculture, what are common examples of agricultural branches.
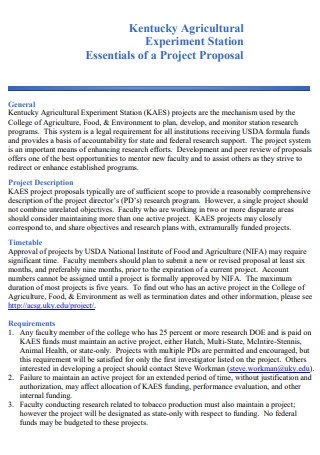
Agricultural Experiment Station Project Proposal
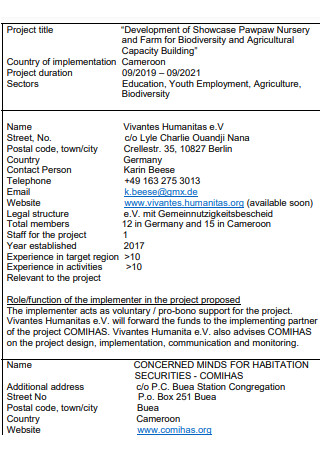
Farm for Biodiversity and Agricultural Project Proposal
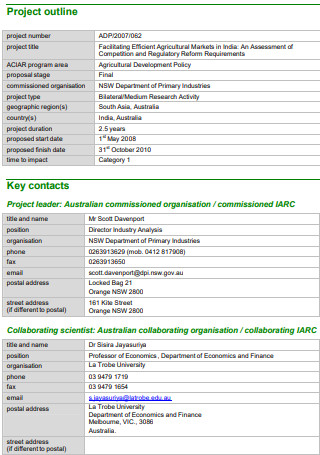
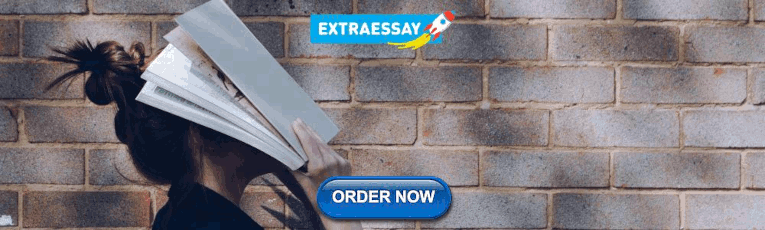
Facilitating Efficient Agricultural Market Project Proposal
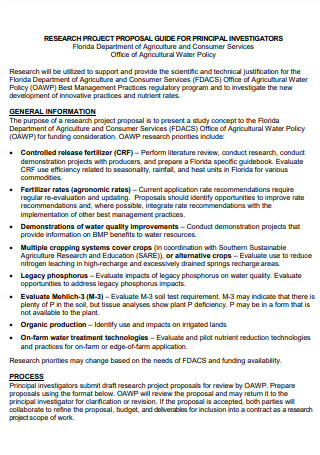
Agricultural Research Project Proposal
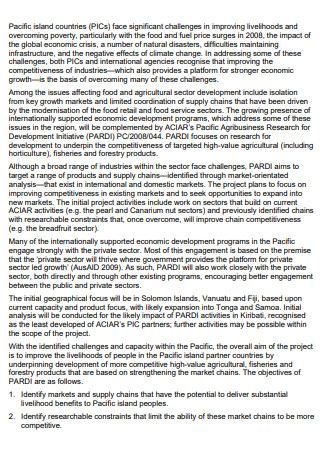
Agricultural Research Agribusiness Project Proposal
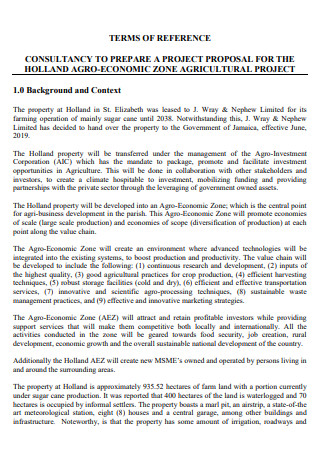
Agroclimatic Zone Agricultural Project Proposal
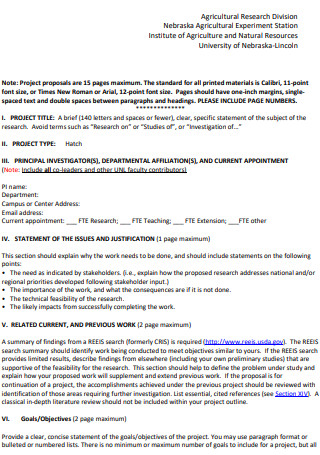
Agricultural Research Division Project Proposal
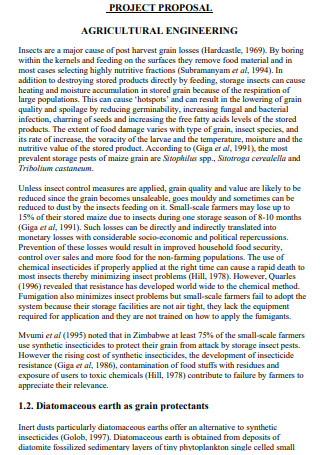
Agricultural Engineering Project Proposal
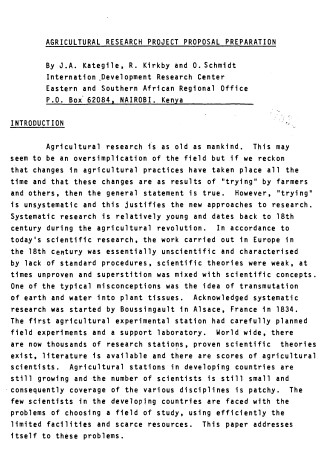
Agricultural Research Project Proposal Preparation
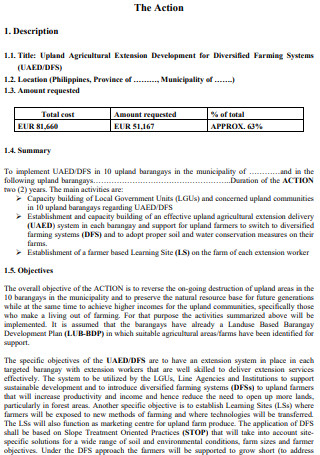
Upland Agricultural Extension Project Proposal
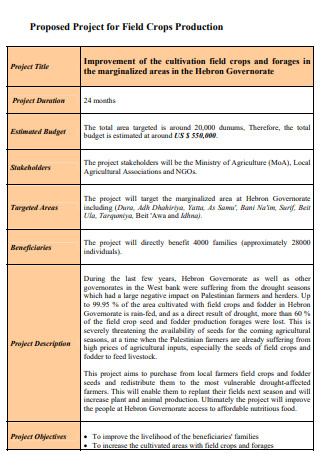
Agricultural Field Crop Production Project Proposal
Tip 1: identify the main problem, tip 2: solution, tip 3: resources and budget, tip 4: timeline, tip 5: agricultural method, share this post on your network, file formats, word templates, google docs templates, excel templates, powerpoint templates, google sheets templates, google slides templates, pdf templates, publisher templates, psd templates, indesign templates, illustrator templates, pages templates, keynote templates, numbers templates, outlook templates, you may also like these articles, 25+ sample construction company proposal in ms word.
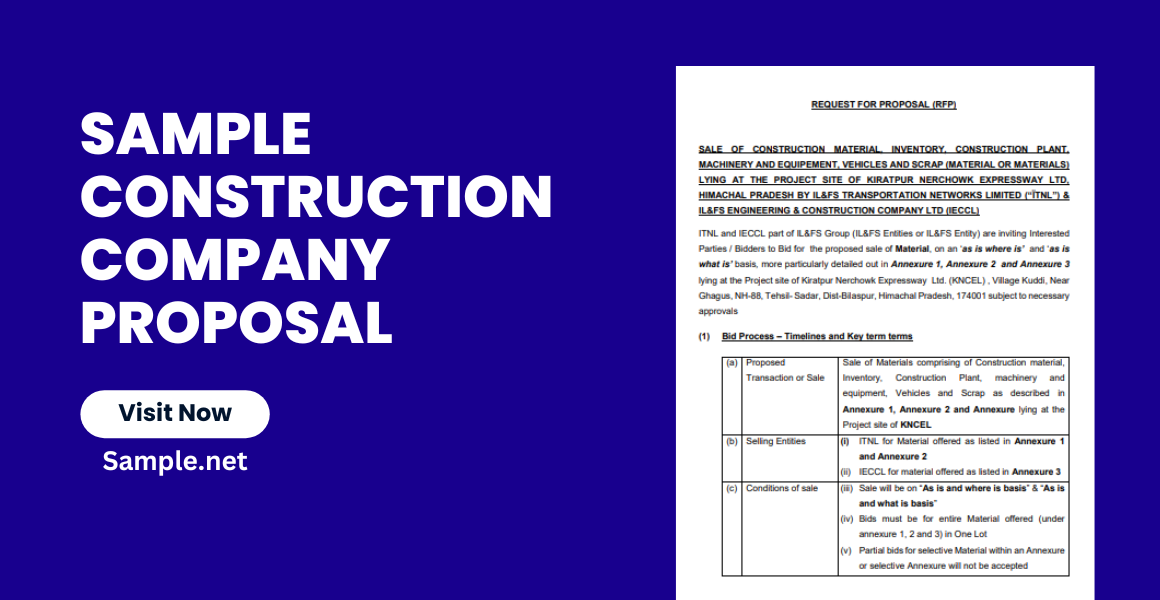
Navigating the intricate world of construction demands a seasoned company with a proven track record. Our comprehensive guide on the Construction Company Proposal is your blueprint to understanding the…
8+ SAMPLE Drama Proposal in PDF
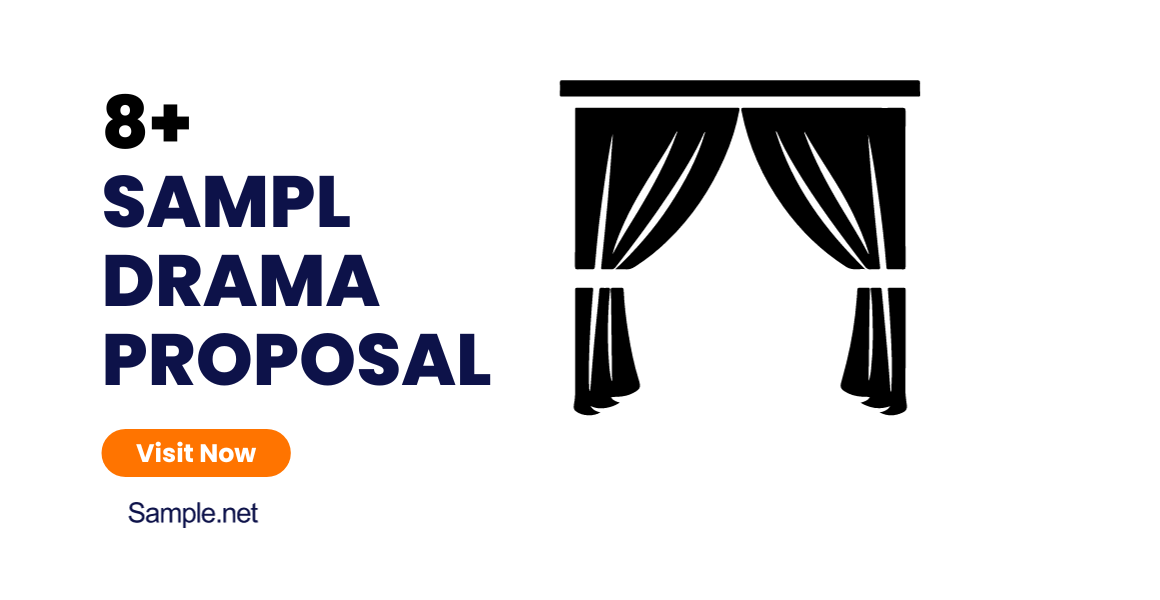
Julia Child said: “Drama is very important in life: You have to come on with a bang. You never want to go out with a whimper. Everything can have…
browse by categories
- Questionnaire
- Description
- Reconciliation
- Certificate
- Spreadsheet
Information
- privacy policy
- Terms & Conditions
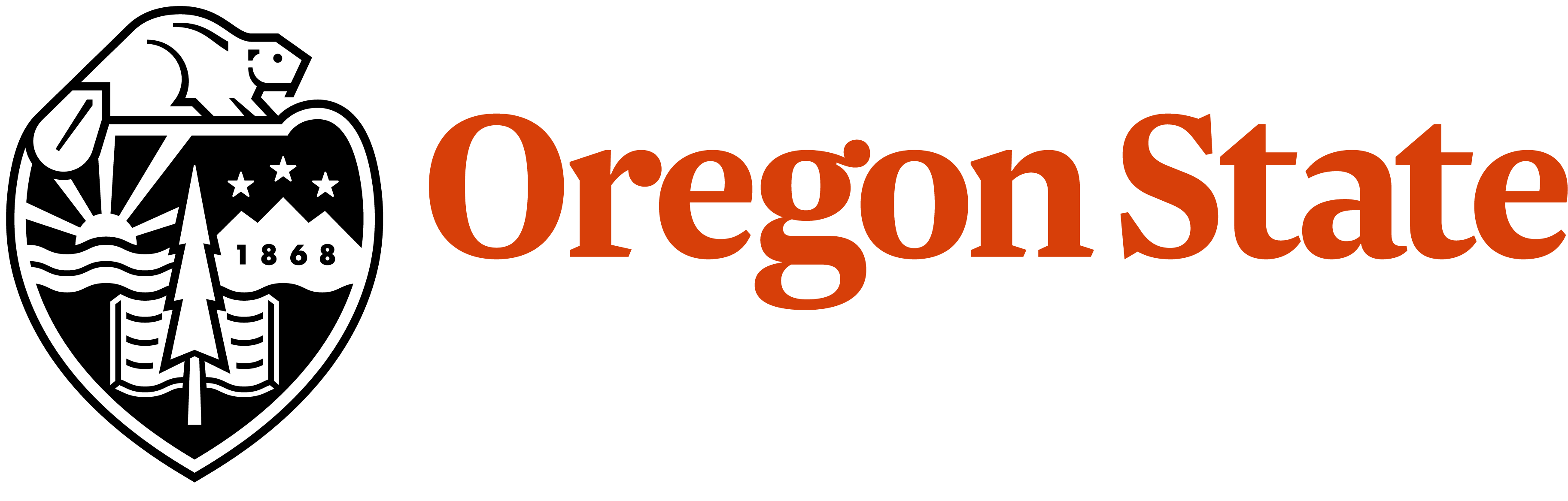
Apply   MyCAS
- Oregon Grass and Legume Seed Production
- 2009 Seed Production Research Report
- 2010 Seed Production Research Report
- 2011 Seed Production Research
- 2013 Seed Production Research Report
- 2014 Seed Production Research Report
- 2015 Seed Production Research Report
- 2016 Seed Production Research Report
- 2017 Seed Production Research Report
- 2018 Seed Production Research Report
- 2019 Seed Production Research Report
- 2020 Seed Production Research Report
- 2021 Seed Production Research Report
- 2022 Seed Production Research Report
2023 Seed Production Research Report
- Seed Production Research Reports
- Comparative Efficacy of Pyrethroid Insecticides Against Symphylans in Tall Fescue Seed Crops
- Industry Survey of Current Practices and Perspectives on Clover Seed Weevil Management in White Clover Seed Production
- Effect of Spring Defoliation and Trinexapac-ethyl (Palisade EC) Plant Growth Regulator on Annual Ryegrass Seed Crops
- Soil Carbon Stock Response to Subsurface Drainage in the North Willamette Valley
- Evaluating Diphacinone and Chlorophacinone Rodenticides in Bait Boxes for Vole Control in Grass Seed Crops
- Lethality of Three Species of Phasmarhabditis Nematodes to Gray Field Slugs in a Microcosm Study
- Preemergent Herbicides for Downy Brome Control in Established Kentucky Bluegrass Grown for Seed
- Residue Management Influence on Downy Brome Control in Established Kentucky Bluegrass Grown for Seed
Author Index
Central oregon agricultural research center—osu.
J.F. Spring, Agronomist, Central Oregon Seeds, Inc. Madras, OR
Department of Crop and Soil Science—OSU
N.P. Anderson, Professor, Extension Seed Production Specialist
B.C. Donovan, Faculty Research Assistant
N. Kaur, Assistant Professor, Extension Entomology Specialist
S. Mahoney, Undergraduate Extnsion Intern
D.J. Maliszewski, Faculty Research Assistant
R.J. Mc Donnell, Associate Professor
C.H. Richart, Faculty Research Assistant
G. Tiwari, Graduate Student
A.A Tomasek, Assistant Professor, Extension Soil and Water Quality Specialist
A.R. Willette, Faculty Research Assistant
Department of Integrative Biology—OSU
D.R. Denver, Professor
D.K. Howe, Senior Faculty Research Assistant
Extension Service—OSU
K.C. Tanner, Field Crops Extension Agent, Linn, Benton and Lane Counties
D.L. Walenta, Extension Agronomist, Union Baker and Wallowa Counties
North Willamette Research and Extension Center—OSU
D.M. Lightle, Assistant Professor (Practice)
National Forage Seed Production Research Center, Corvallis, OR—USDA-ARS
L.C Breza, Postdoctoral Fellow
S.J. Dorman, Research Entomologist
J.M. Moore, Research Soil Scientist
K.M. Trippe, Research Microbiologist
This report has been published with a grant from the Oregon Seed Council
Appreciation is expressed to the Officers of the 2023–2024 Oregon Seed Council:
Kate Hartnell, President Alex Duerst, First Vice President David Goracke, Second Vice President Emily Woodcock, Treasurer Becky Berger, Immediate Past President
Business Address Oregon Seed Council 28415 SW Villebois Dr. N Wilsonville, OR 97070 Tel: (503) 585-1157 E-mail: [email protected] www.oregonseedcouncil.org
Sincere appreciation is also extended to the growers who have allowed trials to be conducted on their farms. Data presented in many of the research reports would not be available without their cooperation.
Lastly, appreciation is expressed to Teresa Welch, Wild Iris Communications, for her conscientious attention to detail in formatting this manuscript for publication.
Tropical Resources Institute
Sample proposal 2, growing food and preserving seeds : ni-vanuatu women’s relationships with food security, biodiversity and food policy, vanuatu, 2012.
PROPOSAL NARRATIVE
Problem Statement, Research Questions, and Research Objectives
More than 1000 varieties of root and tuber crops are grown across a sample group of 10 villages in the island nation of Vanuatu in the South Pacific. Maintaining this diversity is the foundation of the island inhabitants’ food security strategy (CIRAD 2008). Global agricultural and food trade policies are fostering the dominance of cash crops like cocoa, palm and coffee on small farm holdings, altering tropical cropping systems and shortening fallow periods. Due to these changes, small farm holder communities are confronting issues such as limited access to traditional staple foods, depleted finances caused by buying other more expensive grains from the market, loss of biodiversity, and the disappearance of local customs around the cultivation and preparation of food (ANR 2010). Traditionally Ni-Vanuatu women have been responsible for food cropping while men are involved in cash crop production (FAO 2003).
This ethno-botanical study, conducted in the villages located in the island of Santo in Vanuatu, will describe the farming practices followed by women farmers in farms, home gardens and community spaces and ask the following questions – 1) As primary food croppers do women farmers play a decisive role in the in-situ conservation of indigenous genetic resources, maintaining resilient farming systems, and providing their families with access to a balanced diet 2) How are women’s farming efforts perceived and valued in the male-dominated Ni-Vanuatu rural societies by the community, men, and women themselves - as a domestic chore or as an economic contribution 3) How does gender influence a) access to and control over natural resources, b) differential preferences for selecting and growing crop varieties and c) different roles in food production (Gurung, Thapa and Gurung 2000) and 4) how do these social and cultural dynamics shape the gender sensitivity of policies and programs targeted at sustainable agro-development and building food security in Vanuatu.
Literature Review
“ With equal access to productive resources and services, such as land, water and credit, women farmers can produce 20 to 30 percent more food, enough to lift 150 million people out of hunger”, (Diouf 2011). The preservation of biological diversity and plant genetic resources is key to food security. Women are responsible for providing their families with food and care and therefore have specialized knowledge of the value and diverse uses of plants for nutrition, health and income. Consequently they are often the preservers of traditional knowledge of indigenous plants (FAO 1998). Moreover, women often experiment with and adapt indigenous species and thus become experts in plant genetic resources (Karl 1996; Bunning and Hill 1996).
Despite widespread acknowledgment of the critical role that women play in sustainable agriculture and food security in developing countries, lack of synthesized data and analysis of their influence, cultural biases and gender constraints under-value and under-represent their contribution (Villarreal 2010). Much of women's work remains “invisible” (Quisumbing 1998). This lack of recognition of their efforts means that their interests and demands are often overlooked and women are excluded as an active partner and stakeholder in sustainable agro- development. For e.g., in Cameroon, an irrigated rice project did not assign women land because women were expected to work in their husbands’ fields (Quisumbing and Pandolfelli 2008).
The over-arching objective of this research using the context of women farmers in Santo Island in Vanuatu is to study if, and how women farmers contribute to the preservation of genetic resources, the cultivation of a wide diversity of crops and therefore enhance food security for small farm holder communities in developing countries. And at the same time review available evidence and documented studies that assist in understanding the representation and participation of Ni-Vanuatu women in food security and agro-development policy and decision making.
Field Site Selection and Justification
Subsistence agriculture and local food production is vital in the Pacific Islands (IPCC 2007). That small islands have high ecological dependency is well recognized (ADB 2004) with the dependence on plant genetic resources for food cropping can be as high as 80% in some island states with it being 35% in Vanuatu (Ximena 1998). Projected impacts of international food trade and climate change will hamper Vanuatu’s food security (IPCC 2007, ANR 2010). Ni-Vanuatu women are largely responsible for producing food. On average, women spend 30 percent of their time on food production (Agricultural Census, 1983-84). Yet, given the subordinated status of women in Vanuatu's culture, women's role in agriculture is not recognized in many policies, including delivery of extension services (FAO 2003). This makes the villages in the island of Santo, largely the most important farming community in Vanuatu and the principal supplier of fruits and vegetables to the Port Vila market, an ideal location for my research which looks for direct linkages between women, the preservation of bio-diversity in the form of plant genetic resources, and food security, and its subsequent representation in agricultural policy.
Methodology
My overall goal at the end of this summer is to publish a policy proposal that documents empirical evidence, data and observations from my field work and contributes suggestive policies that could help realize the rights of women farmers in policies and programs focused on building food security and preserving bio-diversity across the developing countries.
Through an in-depth field research conducted in Santo Island, Vanuatu, my goal is to gather qualitative observations and quantitative data points around these themes. Research methods include ethnographic study, unstructured interviews, oral narratives and participant observation. The first few days will be spent in familiarizing myself with research groups who are already working in these villages, identifying the villages/farm sites for fieldwork, sensitizing myself to the cultural and social practices of the communities, explaining my presence to the community’s leaders and identifying collaborators. Quantitative questions include the extent of loss of bio- diversity by cash-cropping, how much time is spent on farming for commercial purposes, how much in farming for household production, how many different crops are planted and how many different varieties, what share of natural resources is available to women for food production vis-à-vis men etc. Qualitative aspects include the traditional practices followed in preserving seeds and planting material, the community's and women's perception of their role in securing food for the family, bio-diversity management and their representation in agro-development policies, and how they are adapting their traditional practices to new environmental and socio-political changes.
Personal Qualifications and Research Collaborations
Personal qualifications include past work done with a co-operative to support women farmers in southern India on similar issues, undergraduate and graduate level skills in research methods, and pertinent coursework at Yale specifically Social sciences theory and method, international environmental policy and governance, and environmental protection law. I have been in touch since mid-December with the French research organization CIRAD and VARTC (Vanuatu Agriculture Research and Training Centre) who are working in Santo. Dr. Vincent Lebot, the head of the CIRAD Vanuatu center, well known geneticist and expert on plant breeding in the Pacific Islands, has agreed to supervise my work. Official languages of Vanuatu are English, French and Bislama. I spoke beginner level French, have been taking French classes since the first term and am currently enrolled in Intermediate level French at Yale. French and colloquial English are spoken in the villages where I will be working.
RESEARCH SCHEDULE
February-May
- Confirm contacts, secure funds and plan on the ground arrangements
- Travel logistics
- Continue literature review, prepare questionnaires, work on research methods
- Obtain approval from the Yale Human Subjects Committee
- 1 week pilot survey, finding a guide and/or collaborator, appreciation of Bislama and introduction to the communities
- Interviews – Quantitative Questions
July-August
- Participant observations, quantitative with ethno botanical focus
- Participatory Work, Qualitative study, policy reviews and examination
August-September
- Write and finalize a paper
Academia.edu no longer supports Internet Explorer.
To browse Academia.edu and the wider internet faster and more securely, please take a few seconds to upgrade your browser .
Enter the email address you signed up with and we'll email you a reset link.
- We're Hiring!
- Help Center
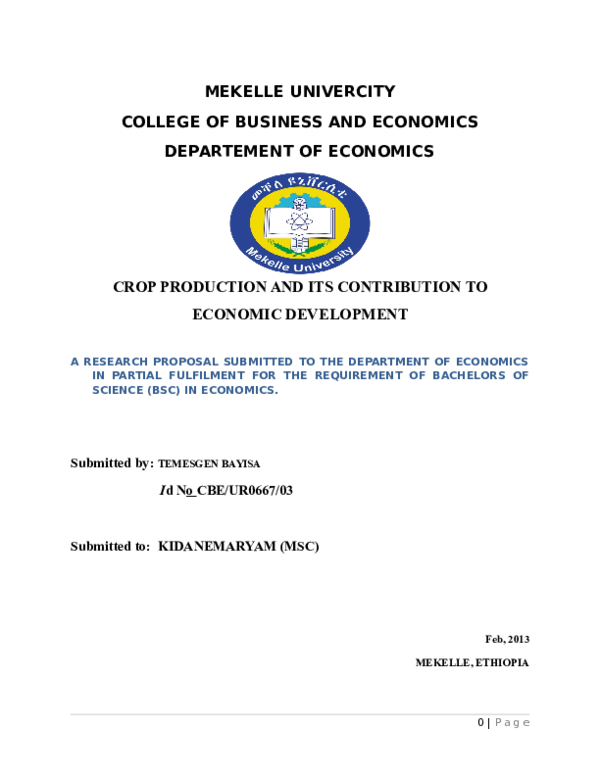
DETERMINANTS OF CROP PRODUCTION AND ITS CONTRIBUTION TO ECONOMIC DEVELOPMENT

ABTSTRACT This paper compute the cost and returns crop productivities and its micro level contribution to income and employment. Besides to this using a multiple linear regression model, empirically examined the factors that determine the crop productivities among farm households. A one year cross sectional data were used. The data were collected from randomly selected farm households in aynalem tabia through scheduled survey questionnaire. Nine variables were entered into the regression model and four variables were found to be statistically significant at less than 5% level of significance. These variables are land size, family labor, amount of fertilizer used and access to extension service. Thediagnostic test of multcollinearity, heteroscedasticity and model specification was carried out. The result of the test shows that the model is free from these problem.
Related Papers
International Journals of Marketing and Technology
Dr. Thirumagal Pillai
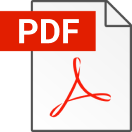
temesgen B bayisa
abrha bihon kassa
Developing Country Studies
getachew tadesse
Sisay Asefa
Diah ayu Novitasari
Abstrak Agriculture in Indonesia is the most important factor in economic development, agriculture problems can be caused by agriculturally functional agricultural lands to industries and settlements, less modern technologies, as well as labor issues that can affect per capita labor income. But it can all be overcome by the large production of agricultural products, this will result in prosperity can be met. The analysis used in this study using MANOVA analysis where researchers will see Testing the correlation between response variables, estimating parameters, Tests of parameter significance. This research was conducted in 1986-2016 macroeconomic data. On multivariate linear regression analysis because the response variable Y1 is Production and Y2 Welfare is dependent or correlated. Variable Harvest Area in general gives a significant effect on the amount of production and welfare. Similarly, the number of workers also gives a significant impact on the amount of production and welfare. However, variable use of technology did not have a significant effect on the amount of production and welfare.
European journal of business and strategic management
Eucabeth Majiwa
International Journal of Research in Social Science and Humanities
robert podstawski
anas I S W A N T O anwar
This study aims to examine agricultural sector budget policies, fertilizer subsidies and irrigation infrastructure that affect farmers' income through the variable productivity of agricultural land in the food crops sub-sector in the 8 districts of Bosowasipilu which were food buffer advocates in South Sulawesi during the 2014-2018 period. Qualitative and quantitative analysis is carried out to illustrate the relationship between land productivity and farmers' income. The Cobb-Gouglas production function is applied by multiple regression models using path analysis for policies that affect the production and productivity of the agricultural sub-sector of rice. The results show that only irrigation infrastructure policies that have a significant effect on land productivity directly, however, do not have a direct effect on farmer's income but through land productivity as an interpening variable, it has a significant effect on farmer's income.
International Journal of Research in Social Sciences
The main thrust of this paper is to examine the production conditions in Indian agriculture. It is expected economic reforms in 1990s for the agrarian sector must have motivated the farmers for a better performance in crop production1. The neo-classical analysis of market behavior cannot be directly applied to study agricultural production. Existence of market imperfections is the important characteristic of the agricultural sector and farmers very often have to plan and carry out their production decision in peculiar complicated conditions. A number of factors influence farmers‟ productions decisions as well as choice of crops and crop combinations. Household resource endowment in terms of land, labor and capital, availability of input and technology, etc., are the major factors involved in decision making. Household food and food security are also important considerations. Another set of factors is the incidence of insect, pests and diseases (witnessed in the recent past), rainfall uncertainty, and soil conditions. Among the more fundamental structure and institutional factors are the availability of infrastructure and marketing support. Furthermore, the degree of price response influences the farmer producing for the market and those engaged in subsistence farming. The main objective of this present study is to examine the determinants of output in a post-reform period.
RELATED PAPERS
edwin orlando pazuña guanoluisa
Norba. Revista de Arte
FEDERICO L. SILVESTRE
MUHAMMED HARIF
Revista ALMUDÍ, Nº 13
Teresa Jular
Mostafa Shahriari
Rachel Darnley-Smith
MRS Proceedings
Hanako Yashiro
International Journal of Electrical and Computer Engineering (IJECE)
Rifqi kurniawan
Nizhny Novgorod Linguistics University Bulletin
Olga Baykova
Romanian Journal of Legal Medicine
Biagio Solarino
Anales De La Facultad De Medicina
Carlos Sevilla
Investigative Ophthalmology & Visual Science
Journal of Labelled Compounds and Radiopharmaceuticals
Dhimant Desai
m amin Sunarhadi
Malihatus Syarifah
Journal of Fundamentals of Renewable Energy and Applications
Journal of Molecular and Cellular Cardiology
Estrella Hernandez
Frontiers in Immunology
Sarah Anzick
IFAC Proceedings Volumes
Oliver Straeter
Fertility and Sterility
Jamie Grifo
International Journal of Advanced Research in Science, Communication and Technology
Umesh Gadekar
Laura Rodriguez
European Journal of Obstetrics & Gynecology and Reproductive Biology
Mandy Caruana
Ana Lía Rey
- We're Hiring!
- Help Center
- Find new research papers in:
- Health Sciences
- Earth Sciences
- Cognitive Science
- Mathematics
- Computer Science
- Academia ©2024
Thank you for visiting nature.com. You are using a browser version with limited support for CSS. To obtain the best experience, we recommend you use a more up to date browser (or turn off compatibility mode in Internet Explorer). In the meantime, to ensure continued support, we are displaying the site without styles and JavaScript.
- View all journals
- My Account Login
- Explore content
- About the journal
- Publish with us
- Sign up for alerts
- Open access
- Published: 26 April 2024
Current and future perspectives for biomass waste management and utilization
- Ornella Francioso 1
Scientific Reports volume 14 , Article number: 9635 ( 2024 ) Cite this article
Metrics details
- Geochemistry
The collection is dedicated to the conversion of biomass wastes into value-added bioproducts and bioactive compounds, with a focus on their applications in the agro-energy sector. Of particular relevance were three studies evaluating the effects of livestock waste, rice straw and biochar on soil properties, crops and productivity. Of note were two articles on the bioconversion of aquaculture sludge by insects and the production of biofuel from seed oil as an alternative to overcoming the depletion of fossil fuels. Finally, one article analysed the potential for recovering organic and mineral compounds from gastropod shells. The articles provided insights into the management and use of biomass wastes, as well as suggestions for future research to promote sustainability in agriculture.
Due to the COVID-19 pandemic and ongoing conflicts, there are rapid and unprecedented changes occurring in the climate, environment, and economy. Therefore, it is necessary to find immediate solutions to these challenges. The goal is to identify and co-develop innovative circular solutions that promote environmental sustainability, resilience, and economic growth in the near future. Agriculture has traditionally operated as a circular economic system, utilizing livestock farming yields waste as manure, animal bedding, and related by-products and crop residue 1 to restore soil fertility. This has been achieved through the use of elemental agricultural practices, taking into account the availability of biomass waste. Advances in knowledge, technology, and synthetic products have significantly increased primary productivity, food production processes, and food security. As a result, the circularity has been lost or reduced, resulting in a return to a linear economic model with a considerable accumulation of waste 2 . Mismanagement of biomass waste has significant environmental impacts due to the GHG emission, pollutants, and unpleasant odours. According to Gerber et al. 3 , agricultural and livestock by-products are responsible for 40% of global CH 4 emissions, while waste disposal accounts for 18% 3 . Therefore, managing biomass waste properly is crucial in mitigating its impact on the climate and environment 4 . The 4Rs, Reduce, Reuse, Recover, and Recycle 5 became mandatory. By using biomass waste, local communities can increase their income, reduce the financial burden of waste disposal and minimize their carbon footprint 6 .
Article highlights in this collection
This Collection focuses on the conversion of biomass waste into value-added bio-products and bioactive compounds, and their applications in the agro-energy sector. The ultimate aim is to reduce waste and promote a sustainable and environmentally friendly approach. Three studies analyzed the effects of livestock waste, rice straw 7 , 9 and biochar 10 on various soil characteristics, crops and productivity. Two papers are presented focusing on how insects bio-convert aquaculture sludge into nutrients 11 , and finding alternative energy sources to overcome the fragility of fossil fuels and their negative environmental effects 12 . Finally, in one paper, the potential for recovering organic and mineral compounds from gastropod shells was explored 13 .
Specifically, Wang et al. 7 investigated the recycling of P from livestock waste, including poultry manure (PM), cattle manure (CM), cattle bone meal (CB), maize straw crop residues (MS), and superphosphate (SSP), in acidic (red soil) and alkaline (river soil) soils that received the same total P supply. The study addressed the problem of low P availability in these soils, which is a major constraint to sustainable crop productivity. Only CM increased soil labile P fractions to levels similar to SSP. These results have important implications for sustainable agriculture, as phosphate fertilizers are produced from phosphate rock, a non-renewable resource 8 .
Ghosh et al. 9 used rice straw at 12 Mg ha −1 combined with P-solubilizing microorganisms and 75% P mineral to improve silicon (Si), organic acid levels, soil enzyme activity and grain yield. The results showed that grain yield increased by 40% compared to no P application, and by 18% compared to 100% P application. Therefore, recycling agricultural residues with phosphorous-solubilizing microorganisms has the potential to increase crop yields and save 25% of mineral P, leading to sustainable soil management.
Pinnavaia et al . 10 addressed the impact of biochar in agriculture. They evaluated the effects of sugarcane bagasse biochar on the growth of cotton ( Gossypium hirsutum L.), as well as the yield and quality of the lint, in a three-year field experiment. Despite the high concentration of biochar added to the soil, no significant effect on cotton lint and seed yield was observed in the first two years. The agricultural and environmental implications of this research are significant, as it highlights the potential of biochar to promote sustainability in agriculture.
An interesting way to promote the transition to a circular economy is to use insects to bio-convert aquaculture sludge to recover nutrients that can be used as animal feed. In addition, the residual material can be conveniently used as organic fertilizer. Rossi et al. 11 used black fly larvae ( Hermetia illucens ) to treat aquaculture sludge waste at different amounts. Overall, this study highlights the potential of Hermetia illucens larvae in managing fresh aquaculture sludge waste, providing a dual benefit of waste reduction and insect biomass production.
Jan et al. 12 studied a new method for producing biofuel from Sisymbrium Irio L. seed oil using a titania catalyst. The authors highlight the significance of this plant as it can be grown in unfavourable environments and, due to its physicochemical properties, produces an eco-friendly fuel that is a competitive source for commercial biodiesel production.
Elegbede et al. 13 investigated the composition of gastropod shells ( Tympanotonus fuscatus , Pachymelania aurita , and Thais coronata ) as a way to recover CaCO 3 and other organic molecules from readily available biomass waste. The use of these materials has proven to be essential and cost-effective in promoting a circular bio-economy.
The articles presented valuable insights into biomass waste management and utilization, as well as suggestions for future research to promote sustainability in agriculture.
Muhammad, S. et al. Insights into agricultural-waste-based nano-activated carbon fabrication and modifications for wastewater treatment application. Agriculture 12 , 1737. https://doi.org/10.3390/agriculture121017372 (2022).
Article CAS Google Scholar
Barros, M. V., Salvador, R., de Francisco, A. C. & Piekarski, C. M. Mapping of research lines on circular economy practices in agriculture: From waste to energy. Renew. Sustain. Energy Rev. 131 , 109958. https://doi.org/10.1016/j.rser.2020.109958 (2020).
Article Google Scholar
Gerber, P. J. et al . Tackling Climate Change through Livestock: a Global Assessment of Emissions and Mitigation Opportunities. (Food and Agriculture Organization of the United Nations (FAO), 2013) http://www.fao.org/docrep/018/i3437e/i3437e.pdf .
Phiri, R., Rangappa, S. M. & Siengchin, S. Agro-waste for renewable and sustainable green production: A review. J. Clean. Prod. https://doi.org/10.1016/j.jclepro.2023.139989 (2024).
Dhanya, M. S. Perspectives of agro-waste biorefineries for sustainable biofuels. In Zero Waste Biorefinery. Energy, Environment, and Sustainability (eds Nandabalan, Y. K. et al. ) 207–232 (Springer, 2022).
Google Scholar
Amran, M. A. et al. Value-added metabolites from agricultural waste and application of green extraction techniques. Sustainability 13 , 11432. https://doi.org/10.3390/su132011432 (2021).
Wang, Y. et al. Soil phosphorus availability and fractionation in response to different phosphorus sources in alkaline and acid soils: a short-term incubation study. Sci. Rep. 13 , 5677. https://doi.org/10.1038/s41598-023-31908-x (2023).
Article ADS CAS PubMed Central Google Scholar
Cordell, D., Rosemarin, A., Schröder, J. J. & Smit, A. L. Towards global phosphorus security: A systems framework for phosphorus recovery and reuse options. Chemosphere 84 , 747–758. https://doi.org/10.1016/j.chemosphere.2011.02.032 (2011).
Article ADS CAS Google Scholar
Ghosh, A. et al. Rice residue promotes mobilisation and plant acquisition of soil phosphorus under wheat ( Triticum aestivum ) rice ( Oryza sativa ) cropping sequence in a semi-arid Inceptisol. Sci. Rep. 13 , 17545. https://doi.org/10.1038/s41598-023-44620-7 (2023).
Pinnamaneni, S. R. et al. Effect of continuous sugarcane bagasse-derived biochar application on rainfed cotton ( Gossypium hirsutum L.) growth, yield and lint quality in the humid Mississippi delta. Sci. Rep. 13 , 10941. https://doi.org/10.1038/s41598-023-37820-8 (2023).
Rossi, G. et al. Fresh aquaculture sludge management with black soldier fly ( Hermetia illucens L.) larvae: investigation on bioconversion performances. Sci. Rep. 13 , 20982. https://doi.org/10.1038/s41598-023-48061-0 (2023).
Jan, H. A. et al. Biodiesel production from Sisymbrium irio as a potential novel biomass waste feedstock using homemade titania catalyst. Sci. Rep. 13 , 11282. https://doi.org/10.1038/s41598-023-38408-y13 (2023).
Elegbede, I. O. et al. Proximate, minerals, carotenoid and trypsin inhibitor composition in the exoskeletons of seafood gastropods and their potentials for sustainable circular utilisation. Sci. Rep. 13 , 13064. https://doi.org/10.1038/s41598-023-38345-w (2023).
Download references
Acknowledgements
I would like to express my gratitude to the authors for their contributions to this Collection, as well as to the Editors and the journal for their support and for providing me with this opportunity.
Author information
Authors and affiliations.
Department of Agricultural and Food Sciences, Alma Mater Studiorum, University of Bologna, Viale G. Fanin 40, 40127, Bologna, Italy
Ornella Francioso
You can also search for this author in PubMed Google Scholar
Corresponding author
Correspondence to Ornella Francioso .
Ethics declarations
Competing interests.
The author declares no competing interests.
Additional information
Publisher's note.
Springer Nature remains neutral with regard to jurisdictional claims in published maps and institutional affiliations.
Rights and permissions
Open Access This article is licensed under a Creative Commons Attribution 4.0 International License, which permits use, sharing, adaptation, distribution and reproduction in any medium or format, as long as you give appropriate credit to the original author(s) and the source, provide a link to the Creative Commons licence, and indicate if changes were made. The images or other third party material in this article are included in the article's Creative Commons licence, unless indicated otherwise in a credit line to the material. If material is not included in the article's Creative Commons licence and your intended use is not permitted by statutory regulation or exceeds the permitted use, you will need to obtain permission directly from the copyright holder. To view a copy of this licence, visit http://creativecommons.org/licenses/by/4.0/ .
Reprints and permissions
About this article
Cite this article.
Francioso, O. Current and future perspectives for biomass waste management and utilization. Sci Rep 14 , 9635 (2024). https://doi.org/10.1038/s41598-024-59623-1
Download citation
Published : 26 April 2024
DOI : https://doi.org/10.1038/s41598-024-59623-1
Share this article
Anyone you share the following link with will be able to read this content:
Sorry, a shareable link is not currently available for this article.
Provided by the Springer Nature SharedIt content-sharing initiative
Quick links
- Explore articles by subject
- Guide to authors
- Editorial policies
Sign up for the Nature Briefing newsletter — what matters in science, free to your inbox daily.

Improving Decision Support Tools for Quantifying GHG Emissions from Organic Production Systems
- Open access
- Published: 26 April 2024
Cite this article
You have full access to this open access article
- Meagan E. Schipanski ORCID: orcid.org/0000-0002-1661-9858 1 ,
- Shelby C. McClelland ORCID: orcid.org/0000-0002-1827-3029 2 ,
- Helen M. Hughes ORCID: orcid.org/0000-0002-9704-8080 3 ,
- Randa Jabbour ORCID: orcid.org/0000-0001-8230-9447 4 ,
- Daniella Malin 5 ,
- Jonathan Hillier 4 ,
- Keith Paustian 1 &
- Elizabeth Reaves 5
As food companies have adopted sustainability metrics to quantify the environmental impacts of supply chains, we need data-driven decision support tools that represent organic management practices. Decision support tools such as COMET-Farm and the Cool Farm Tool have been developed to estimate management practice impacts on soil carbon and greenhouse gas emissions from agricultural systems, but these tools have primarily been developed and used to evaluate conventional management systems. We provide an overview of the research, outreach, and educational activities used to improve these tools to better integrate organic management practices with a focus on cover crops. We summarize our previously published findings from a meta-analysis of the average potential soil carbon benefits of cover crops in temperate climates that identified planting window, biomass production, and soil texture as important predictors of cover crop soil carbon outcomes. We demonstrate how these findings were applied to improvements in process-based models and the parameterization of empirical models. In addition, we solicited feedback from organic community members on the utility of these tools and identified barriers to adoption. Finally, we evaluated both tools as resources for teaching undergraduate students about organic management systems and their impacts on greenhouse gas emissions. While both tools contain a range of customizable, organic amendment options, grazing management options still need further improvement. These improved decision support systems can help identify opportunities for enhancing the sustainability of organic systems.
Avoid common mistakes on your manuscript.
Introduction
Organic foods have gained broad consumer support due to expectations that organic production systems provide healthier food and support healthier ecosystems. However, environmental benefits are not universally realized and there are increasing calls for agricultural systems to meet quantitative sustainability metrics. The number of supply chain sustainability metrics increased from less than 10 to more than 130 between 1999–2012 (Ahi and Searcy 2015 ) and have only continued to expand since then. North American food companies are developing their own supply chain metrics and rating systems, such as the Whole Foods rating system for fresh produce and the Field to Market Fieldprint calculator. In addition, voluntary carbon markets are bringing more attention to the development of agriculturally-based offsets, particularly two of the largest registries in the voluntary market space, Verified Carbon Standard and American Carbon Registry. The increased interest in natural climate solutions and the emergence of carbon markets requires improved estimates of agricultural management impacts on soil carbon (C) stock changes. Organic production practices are not always accurately evaluated within these supply chain rating systems and are not specifically included in offset protocols.
Assessments of the environmental impacts of organic agriculture have focused more broadly on impacts on soil quality, biodiversity, nutrient losses, and reductions in pesticide use while greenhouse gas (GHG) emissions and climate change mitigation have received less attention (Smith et al. 2019 ; Clark 2020 ). A limited number of studies have evaluated the relative GHG emissions from organic production systems relative to conventional management systems. These studies have found varying outcomes though with a general consensus that organic systems tend to have increased soil carbon stocks and reduced nitrous oxide emissions relative to conventional systems on a per area basis (i.e., absolute emissions; Tuomisto et al. 2012 ), but equivalent or higher GHG emissions on a per unit harvested basis (i.e., emissions intensity; Tuomisto et al. 2012 ; Skinner et al. 2014 , 2019 ). However, there is high variability in management practices within organic systems, which can result in contrasting climate impacts and changes in soil C stocks (Gaudaré et al. 2023 ) and less research has focused on identifying management strategies within organic systems that contribute to GHG emission reductions. Given the general lack of a benefit of organic farming on GHG emissions relative to conventional systems, the organic community risks losing consumer trust as a management approach associated with reduced environmental impacts without engaging more actively in identifying management innovations to reduce emissions.
One obstacle for the organic community's engagement in the expanded interest in quantifying natural climate solutions is that organic production practices are not always accurately evaluated within existing GHG emission tools. This is due in part to a lack of data and quantification methods and the oversimplification of management scenarios in decision support tools. Organic production systems rely on a greater understanding and management of natural biological and biogeochemical processes, which can result in a high degree of management complexity. For example, organic systems tend to have more diverse rotations and rely on more variable nutrient sources, such as manures and composts. This complexity is a challenge for quantifying GHG emissions reductions and soil C changes associated with organic systems. For example, the tools do not allow for intercropping and the multi-year impacts of a single application of an organic amendment on greenhouse gas emissions and soil carbon stocks has a higher degree of uncertainty relative to the the impacts of synthetic fertilizer inputs. Many practices used in organic systems are also applicable to conventional agriculture, such as cover cropping. From a conservation perspective, the inclusion of a broader suite of conservation practices in decision support tools can support the wider adoption of climate mitigating practices.
We present a review and synthesis of recent efforts to improve two well-established decision support tools to better represent organic management scenarios. The decision support systems COMET-Farm and the Cool Farm Tool are two of the most commonly used tools adopted for quantifying the impacts of land use and management practices on soil C and GHG emissions from agricultural systems, but using very different approaches and adopted by different food system actors. We provide an overview of each tool and recent efforts to improve their capacity to estimate the soil C benefits associated with common organic practices with a primary focus on cover crops as an example practice. In addition, we outline key priorities for improving the utility of the tools for organic producers seeking to document and communicate the effects of their management decisions on GHG emissions.
Part 1. The tools
The COMET-Farm system ( https://comet-farm.com/ ) was developed as a decision support system to estimate C sequestration and GHG emissions at the farm-scale on a land-area basis for a wide variety of soil conservation practices. It is an online decision support system (Paustian et al. 2002 , 2004 , 2009 , 2017 ) initially developed with support from the U.S. Department of Agriculture, Natural Resource Conservation Service (USDA, NRCS) to estimate GHG emissions reductions associated with the adoption of incentivized conservation management practices and has since expanded over the past 25 years to include forestry and livestock systems while still maintaining a primary focus on the United States. The system includes all GHG source and removal categories for carbon dioxide (CO 2 ), nitrous oxide (N 2 O) and methane (CH 4 ). Primary users of the system originally included NRCS field staff, emission offset project developers, researchers and students. The COMET-Farm system is a free, web-based platform that combines a fully spatial graphical user interface (GUI) with an extensive set of spatial and tabular databases and a suite of GHG emission models (Fig. 1 ). The system implements USDA entity-scale methods for GHG inventory in agriculture and forestry (Eve et al. 2014 ). This document lays out the methods implemented in COMET-Farm that consist of a variety of both empirical and process-based models for all significant emission sources.
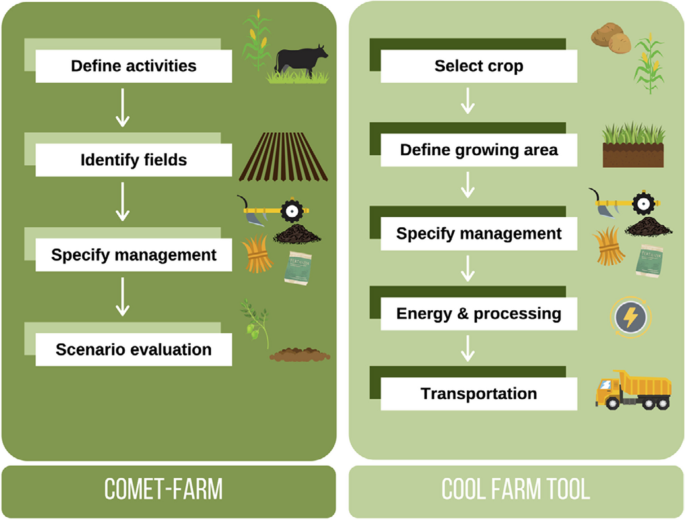
Schematic of the information inputs and supply chain components included in each tool. Adapted from Jabbour et al. 2021
The COMET-Farm tool utilizes the process-based, ecosystem model, DayCent (Parton et al. 1998 , Del Grosso et al. 2010 , Parton et al. 2015 ), that simulates biomass and soil C and N dynamics, including trace gas emissions of N 2 O and CH 4 from soils and CH 4 oxidation in soil. Submodels for plant growth and vegetation dynamics provide capabilities to model annual and perennial crops, grasslands, forest and agroforest systems. The model also includes a full water balance model that operates at a daily time step and includes all major water balance components, including precipitation, irrigation, interception, snow storage and melt, infiltration, runoff, saturated and unsaturated flow, drainage and water storage in up to 20 vertically distributed soil layers. Hence, COMET-Farm can also inform producers on improving water use efficiency and reducing water consumption and nitrate leaching.
The COMET-Farm framework is designed for use by farmers, crop consultants, or others who are not specialists on GHGs but who know the management practices being performed on their land. The GUI provides simple pull-down menu choices that allow the user to enter detailed management information at the individual field scale. It includes built-in mapping function that allow the user to delineate fields or management zones and then can specify crop rotations, tillage, fertilization, irrigation, manuring and organic amendments, harvest, residue management and other practices with a high degree of flexibility. The system also links dynamically to the USDA-NRCS Soil Datamart web-service to extract SSURGO-scale soil property data to use in the model. Model runs are then generated automatically for each soil map unit within each field, and then compiled into on-line and downloadable reports with tables and graphics.
The Cool Farm Tool (CFT) ( http://coolfarmtool.org ) is an online calculator that enables farmers and food supply chain companies to estimate GHG emissions, and understand mitigation options for agricultural production. In particular, it was developed starting in 2008 to assist food companies in setting GHG emission benchmarks and quantifying progress in achieving GHG reduction targets. The tool is free to use by individual farmers. Commercial use by supply chain businesses requires a user membership fee. The web tool is based on an Microsoft Excel model originally created by the University of Aberdeen in partnership with Unilever and the Sustainable Food Lab (Hillier et al. 2011 ). The Cool Farm Tool works by providing users with the ability to enter soil and climate characteristics along with agricultural production practices associated with a given crop or food product. For example, the Cool Farm Tool was used by Costco to quantify GHG emissions from their entire supply of domestic organic eggs from 2010 to 2012 (Vetter et al. 2018 ). Every farmer serving each region of the country participated– representing production of over 1.5 billion eggs.
Originally initiated by Unilever, a number of the world’s largest food and drink businesses and NGOs now cooperate in the Cool Farm Alliance, a membership organization dedicated to maintaining and improving the Cool Farm Tool and spreading the use of the Tool in order to drive emissions reductions from global agriculture. The Gold Standard Foundation has partnered with the Cool Farm Alliance to design methodologies using the Cool Farm Tool and Soil Capitol uses the Cool Farm Tool to enable farmers to earn extra income by providing “insets” or Scope 3 GHG emissions reductions as an added incentive to implementing agricultural practices that reduce GHG emissions and sequester carbon in soils and biomass.
In contrast to COMET-Farm, the Cool Farm Tool calculates emissions using empirical models (Table 1 ). As more data become available, the models can be improved and refined based on the new research findings. Emissions are reported for CO 2 , CH 4 , and N 2 O for the major sources of on-farm emissions associated with the production of agricultural products. Currently the IPCC 2019 Tier 1 model – with enhancements that capture more site specific moisture information—is used to model N 2 O emissions (IPCC 2019 ). In temperate climate zones, for non-rice crops, the wet/dry definition is not determined by the annual climate zone. Instead, it considers whether the weather is wet or dry 30 days before and after the date of fertiliser application, i.e. 60 days in total. This timeframe accounts for the fact that a significant portion of emissions occurs shortly after fertiliser application. For estimating soil C stock changes (and hence net CO 2 emissions or removals), the model is derived from the Tier 1 IPCC 2019 method. Additional elements have been added to account for changes that only affect a portion of a field, and to factor in a historical measured value.
Part 2. Data synthesis to improve model accuracy
Here we summarize our data synthesis approach and how we then integrated this literature synthesis into improvements for COMET-Farm and the Cool Farm Tool. As a case study practice, we synthesized existing datasets to improve the capacity of both tools to estimate the soil C outcomes of cover crop practices. We selected cover crops due to their broad applicability across all scales and types of farming systems and their demonstrated potential to increase soil C and other soil health indicators (Wood and Bowman 2021 ). Thus, cover cropping is gaining attention for its potential as a natural climate solution yet the impacts vary considerably with initial soil conditions and climate (Vendig et al. 2023 ; Deines et al. 2023 ). Our goal was to identify the sources of variability in cover crop impacts on soil C to improve decision support tools both at the user end (data input requirements) and the underlying process-based and empirical models to improve confidence in tool estimates.
As a first step, we conducted and published a meta-analysis of cover crop studies in temperate climates to quantify the effect of cover crops on soil C stocks from the 0–30 cm soil depth and to identify key management and ecological factors that impact variation in this response (McClelland et al. 2021a ). We conducted a systematic review of the literature and identified 40 unique publications with 181 observations that met our inclusion criteria. These publications were restricted to temperate climates representing six countries across three continents and included both organic and conventional farming systems. We estimated the size of the effect of SOC for each combination of cover crop (treatment) and no cover crop (control) within a study where the only variation across treatments was the presence or absence of a cover crop. All calculations were done using the metafor package (Viechtbauer 2010 ) in R version 3.5.2 (R Core Team 2013 ). See McClelland et al. ( 2021a ) for a full overview of methods.
Briefly summarizing the previously published results from this meta-analysis, we found that inclusion of cover crops in annual and perennial cropping systems increased soil organic carbon stocks from 0–30 cm by 12%, averaging 1.11 Mg C ha −1 more soil C relative to a similarly managed system without cover crops (McClelland et al. 2021a ). We did not find time of cover crop implementation was a useful predictor of SOC stock changes, thus we were unable to assign an annual accrual rate. The variables explaining the most variability in soil C effects of cover cropping were planting and termination date (i.e., growing window), annual cover crop biomass production, and soil clay content. Cover crops planted as continuous cover or autumn planted and terminated led to 20–30% greater total soil C stocks relative to other cover crop growing windows. Likewise, high annual cover crop biomass production (> 7 Mg ha −1 yr −1 ) resulted in 30% higher total soil C stocks than lower levels of biomass production. There were no differences in soil C stock responses to cover crops when management system (conventional or organic) was used as a predictor.
As step two, the dataset developed for the meta-analysis was then applied to inform and improve both the COMET-Farm and Cool Farm Tool. To improve COMET-Farm’s ability to more accurately predict soil C stock changes, we focused on improving the capacity of the DayCent model to simulate cover crop biomass production (McClelland et al. 2021b ). The DayCent model was parameterized for one new cover crop species not previously included as a cover crop option in COMET-Farm, sunn hemp ( Crotalaria juncea ). Model performance was then validated and evaluated for sunn hemp as well as other existing cover crop species, annual ryegrass ( Lolium perenne ssp. multiflorum ), cereal rye ( Secale cereale ), clovers ( Trifolium spp. ), vetch ( Vicia spp. ), and cover crop mixtures using published and long-term experimental cover crop production datasets from North America. As described in McClelland et al. ( 2021b ), while DayCent was able to generally simulate changes in soil C stocks with cover crop adoption, the model varied by cover crop species in its ability to simulate cover crop biomass production, with the best performance for annual ryegrass and sunn hemp and weaker correlations between actual and predicted biomass for the vetch. In addition, the simulations identified limitations in the model’s ability to predict cover crop residue quality (C:N) and nitrous oxide emissions. Through this model improvement process, we identified key data gaps. In particular, it would be beneficial if cover crop studies included more detailed management and performance data, such as stand establishment, pest pressure, and soil nutrient and water availability, that influence cover crop biomass production.
We also utilized the meta-analysis dataset to improve the empirical formulas utilized by the Cool Farm Tool to estimate cover crop impacts on SOC stock changes as described in Hughes et al. ( 2023 ). We applied linear, multiple mixed effects regression models to identify the most parsimonious empirical, regression model for predicting cover crop effects on changes in SOC. Candidate predictor variables included soil bulk density, CC biomass, CC C:N ratio, soil texture, experiment duration, mean annual precipitation, mean annual temperature, soil pH, and tillage. All statistics were conducted using R software (packages included lmer). We evaluated 300 models and retained 10 models with the lowest Akaike Information Criterion ( \(AIC\) , Akaike 1998 ), values which also satisfied \({R}_{m}^{2}\) >0.1 and \({p}_{int}\) < 0.05. Models were compared with the IPCC Tier 1 approach (IPCC 2019 ) and the response ratios from our meta-analysis (McClelland et al. 2021a ).
The most parsimonious model that also performed better than the current IPCC Tier 1 model approach utilized by the Cool Farm Tool, included the predictor of CC biomass. In particular, a CC biomass greater than approximately 1 Mg ha −1 yr −1 was required for a positive change in SOC in surface soils. The Cool Farm Alliance is now considering a revision to integrate these findings as part of a larger update to the Cool Farm Tool methodology, which may include asking users to estimate cover crop biomass productivity by category.
Part 3. Meeting the needs of the organic food sector and organic producers
Given the similar goals but different approaches utilized by these two decision support tools, we conducted a joint needs assessment of organic agriculture community members regarding the COMET-Farm and Cool Farm Tools, including priorities for tool improvement and factors that would increase their utility and adoption. Stakeholders surveyed over the life of the project included organic industry and research professionals, organic food company representatives, undergraduate students in the agricultural sciences, and organic producers. Feedback was solicited through workshop surveys, undergraduate classrooms (Jabbour et al. 2021 ), and project informational meetings and presentations (Table 2 ).
Organic community member answers to survey questions (Table 2 ) varied by potential user type and can broadly be grouped into concerns regarding data confidence or uncertainty, the ability of the tools to accurately reflect farming system complexities, and the effort required by the end user to use the tools.
Food company actors interested in utilizing these tools to quantify and demonstrate improvement in meeting stated supply chain GHG emissions reductions wanted a better understanding of the confidence in model estimates. This focus on improving model confidence has been particularly important as interest and investment in carbon credits has increased in recent years.
Producers and tool users wanted to see increased management options within the tools to better reflect more complex management systems. Examples of desired practices included cover crop companion cropping, intercropping, and grazing. Integrated crop-livestock producers identified several areas for improvement. Livestock systems are currently separate from land use systems within COMET-Farm, which makes it difficult for integrated crop-livestock systems to understand where to input certain variables such as manure. Increased management options would be beneficial to represent varying manure quality throughout the year.
Tool integration
As food companies increasingly try to track Tier 2 supply chain emissions, the burden for data collection increasingly falls to farmers or technical service providers working directly with farmers. In general, GHG decision support tools are more likely to be used when supported by a technical service provider. Neither group, but particularly farmers, are likely to use these tools unless there is a requirement from a customer/buyer or financial incentive. For example, U.S farmers are already required to create nutrient management plans so it would be helpful if GHG decision support tools could be linked to other required reporting programs. The key to increase tool utilization is simplicity and integration with existing farm recordkeeping systems or tools, which may be counter to the simultaneous desire to have these tools better represent the complexity of organic farming systems.
Part 4. Educational applications of decision support tools
An often overlooked, but an equally important potential use of these decision support tools is in formal and informal educational settings. Understanding how agricultural and food systems affect climate change is now a core topic addressed across a wide range of university courses, including agronomy, crop science, agroecology, food systems, and environmental science. However, similar to the organic agriculture research foci mentioned above, university courses focused on organic agriculture tend to prioritize soil fertility, ecological principles, and organic certification standards while climate change receives far less attention (Jabbour and Pellissier 2019 ). In addition, the general public is increasingly concerned about climate change (Thackeray et al. 2020 ) and seeking information about agriculture’s contributions to GHG emissions. Freely available decision support tools, including but not limited to COMET-Farm tool and the COOL FARM TOOL, can be utilized effectively to help students and the general public learn about the impact of different production systems on GHG emissions.
To evaluate the utility of the tools to advance learning about the GHG impacts of various food production systems, we developed student learning activities focused on using COMET-Farm and the Cool Farm Tool in undergraduate classes (Jabbour et al. 2021 ). With COMET-Farm, students were asked to simulate a baseline cropping system and then compare the GHG emissions from that baseline to future scenarios that implemented improved nitrogen management and conservation tillage practices. Students were then asked to interpret the scenario results, including discussing the relative impact of their management scnarios and the primary sources of GHG within the farm system. With the Cool Farm Tool, students were presented with a case study of an organic producer interested in identifying ways to mitigate GHG emissions in her corn production system. Students then compared emissions from different scenarios to identify the management change with the greatest impact.
We found that using both tools encouraged systems-thinking. Students gained a greater understanding of how agricultural practices influence GHG emissions along with several other key concepts. Previous research has shown that utilizing system models uses distinctly different neural pathways than reading about a concept (Clark 2020 ). The tools, particularly in the COMET-Farm case, helped students understand the trade-offs or synergies from implementing different practices such as the impacts of replacing synthetic fertilizers with organic amendments on both N 2 O emissions and soil C sequestration. In addition, sharing with students how these tools emerged to address agricultural business and conservation stakeholder concerns and are being utilized in the real-world, helped link course concepts to their real-world applications. Similarly, in less formal educational settings, stakeholders commented that the best use of these tools is educational more than quantitative because they enable a discussion of the potential impact of different practices that a producer might adopt, especially when used in partnership with technical support.
Our goal with this integrated research and outreach was to enhance the capacity across the organic food sector to evaluate and improve management systems and environmental outcomes. While tools that estimate GHG emissions from different agricultural systems can be used by organic producers, these tools were not developed with the complexity of diverse, organic management systems specifically in mind. Through the use of large datasets, meta-analyses, and regression analyses, we identified the most important management and site characteristic variables likely to best predict the soil C impacts of integrating cover crops within temperate cropping systems. Both statistical approaches highlighted the importance of developing tools and approaches to easily estimate cover crop biomass production for reducing uncertainty in soil C outcomes. Our approach highlights the benefits of collaboration across modeling teams. We were able to use a single data synthesis effort (the meta-analysis dataset) to improve two very different models—one through improvements in a process-based model and the other by improving an empircal modeling approach.
We also integrated a wide range of stakeholders to better understand the barriers and potential opportunities for improving the utility of soil C and GHG decision support tools within the organic agriculture sector. We identified key areas for potential to streamline and clarify management input requirements, particularly for integrated grazing systems, as well as the important role that technical service providers will likely continue to play in assisting producers in utilizing these tools. Across the spectrum from producers to students, these decision support tools can serve as critical educational tools that facilitate systems-thinking and the relative potential benefits and trade-offs of implementing different management practices across a wide range of farming systems.
One important point of note is that our work focused primarily on datasets and stakeholders from temperate, northern hemisphere regions. There is still limited data and, hence, much less confidence in extending these tools for use in more tropical and southern hemisphere regions (Richards et al. 2016 ). However, see more recent publications from the Global South such as Das et al. ( 2022 ). Continued investment and engagement in participatory research and outreach with partners in the Global South is essential for the improvement of tools and more equitable access to corresponding incentive programs for natural climate solutions.
Data availability
Not applicable as the data referenced in this synthesis has been previously published and data is available through those publications.
Ahi P, Searcy C (2015) An analysis of metrics used to measure performance in green and sustainable supply chains. J Clean Prod 86:360–377
Article Google Scholar
Akaike H (1998) Information theory and an extension of the maximum likelihood principle. Selected papers of Hirotugu Akaike. Springer, New York, NY, pp 199–213
Chapter Google Scholar
Clark S (2020) Organic farming and climate change: The need for innovation. Sustainability 12(17):7012
Core Team R (2013) R: a language and environment for statistical computing. R Foundation for Statistical Computing, Vienna, Austria. http://www.R-project.org/
Das S, Chatterjee S, Rajbanshi J (2022) Responses of soil organic carbon to conservation practices including climate-smart agriculture in tropical and subtropical regions: A meta-analysis. Sci Total Environ 805:150428
Article CAS PubMed Google Scholar
Deines JM, Guan K, Lopez B, Zhou Q, White CS, Wang S, Lobell DB (2023) Recent cover crop adoption is associated with small maize and soybean yield losses in the United States. Glob Change Biol 29(3):794–807
Article CAS Google Scholar
Del Grosso SJ, Ogle SM, Parton WJ, Breidt FJ (2010) Estimating uncertainty in N2O emissions from US cropland soils. Global Biogeochem Cy 24:1009
Google Scholar
Eve MD, Pape M, Flugge R, Steele D, Man M, Riley-Gilbert S, Biggar (eds) (2014) Quantifying greenhouse gas fluxes in agriculture and forestry: methods for Entity-Scale Inventory. USDA Office of the Chief Economist, Climate Change Program Office. USDA Technical Bulletin 1939. Downloadable at http://www.usda.gov/oce/climate_change/estimation.htm
Gaudaré U, Kuhnert M, Smith P, Martin M, Barbieri P, Pellerin S, Nesme T (2023) Soil organic carbon stocks potentially at risk of decline with organic farming expansion. Nat Clim Chang 13(7):719–725
Hillier J, Walter C, Malin D, Garcia-Suarez T, Mila-i-Canals L, Smith P (2011) A farm-focused calculator for emissions from crop and livestock production. Environ Model Softw 26(9):1070–1078
Hughes HM, McClelland SC, Schipanski ME, Hillier J (2023) Modelling the soil C impacts of cover crops in temperate regions. Agric Syst 209:103663
IPCC (2019) Refinement to the 2006 IPCC Guidelines for National Greenhouse Gas Inventories. Calvo Buendia E, Tanabe K, Kranjc A, Baasansuren J, Fukuda M, Ngarize S, Osako A, Pyrozhenko Y, Shermanau P, Federici S (eds) Published: IPCC, Switzerland
Jabbour R, McClelland SC, Schipanski ME (2021) Use of decision‐support tools by students to link crop management practices with greenhouse gas emissions: A case study. Natural Sci Educ e20063
Jabbour R, Pellissier ME (2019) Instructor priorities for undergraduate organic agriculture education. Natural Sciences Education 48(1):1–7
McClelland SC, Paustian K, Schipanski ME (2021a) Management of cover crops in temperate climates influences soil organic carbon stocks: a meta-analysis. Ecol Appl 31(3):e02278
Article PubMed Google Scholar
McClelland SC, Paustian K, Williams S, Schipanski ME (2021b) Modeling cover crop biomass production and related emissions to improve farm-scale decision-support tools. Agric Syst 191:103151
Parton WJ, Hartman MD, Ojima DS, Schimel DS (1998) DAYCENT: its land surface submodel: description and testing. Glob Planet Chang 19:35–48
Parton WJ, Gutmann MP, Merchant ER, Hartman MD, Adler PR, McNeal FM, Lutz SM (2015) Measuring and mitigating agricultural greenhouse gas production in the US Great Plains, 1870–2000. Proc Natl Acad Sci 112(34):E4681–E4688
Article CAS PubMed PubMed Central Google Scholar
Paustian K, Brenner J, Easter M, Killian K, Ogle S, Olson C, Schuler J, Vining R, Williams S (2009) Counting carbon on the farm: Reaping the benefits of carbon offset programs. J Soil Water Conserv 64:36A-40A
Paustian KJ, Brenner K, Killian J, Cipra S, Williams ET, Elliott MD, Eve T, Kautza Bluhm G (2002) State-level analyses of C sequestration in agricultural soils. In: Kimble JM, Lal R, Follett RF (eds) Agriculture Practices and Policies for Carbon Sequestration in Soil. Lewis Publishers, CRC Press, Boca Raton, Fl., USA, pp 193–204
Paustian K, Babcock B, Kling C, Hatfield J, Lal R, McCarl B., ... Rosenzweig C (2004) Agricultural Mitigation of Greenhouse Gases: Science and Policy Options. Council on Agricultural Science and Technology (CAST) report, R141 p 120
Paustian K, Easter M, Brown K, Chambers A, Eve M, Huber A., ...Williams S (2017) Field‐and farm‐scale assessment of soil greenhouse gas mitigation using COMET‐Farm. Precision conservation: geospatial techniques for agricultural and natural resources conservation 59:341–359
Richards M, Metzel R, Chirinda N, Ly P, Nyamadzawo G, Duong Vu, Q., … & Rosenstock, T. S. (2016) Limits of agricultural greenhouse gas calculators to predict soil N2O and CH4 fluxes in tropical agriculture. Sci Rep 6(1):26279
Skinner C, Gattinger A, Krauss M, Krause HM, Mayer J, Van Der Heijden MG, Mäder P (2019) The impact of long-term organic farming on soil-derived greenhouse gas emissions. Sci Rep 9(1):1702
Article PubMed PubMed Central Google Scholar
Skinner C, Gattinger A, Muller A, Mäder P, Flieβbach A, Stolze M., ... Niggli U (2014) Greenhouse gas fluxes from agricultural soils under organic and non-organic management—A global meta-analysis. Sci total environ 468:553–563
Smith LG, Kirk GJ, Jones PJ, Williams AG (2019) The greenhouse gas impacts of converting food production in England and Wales to organic methods. Nat Commun 10(1):4641
Thackeray SJ, Robinson SA, Smith P, Bruno R, Kirschbaum MU, Bernacchi C., ... Long S (2020) Civil disobedience movements such as School Strike for the Climate are raising public awareness of the climate change emergency. Glob Chang Biol 26(3):1042–1044
Tuomisto HL, Hodge ID, Riordan P, Macdonald DW (2012) Does organic farming reduce environmental impacts?–A meta-analysis of European research. J Environ Manage 112:309–320
Vendig I, Guzman A, De La Cerda G, Esquivel K, Mayer AC, Ponisio L, Bowles TM (2023) Quantifying direct yield benefits of soil carbon increases from cover cropping. Nat Sustain 6(9);1125–1134
Vetter SH, Malin D, Smith P, Hillier J (2018) The potential to reduce GHG emissions in egg production using a GHG calculator–A Cool Farm Tool case study. J Clean Prod 202:1068–1076
Viechtbauer W (2010) Conducting meta-analyses in R with the metafor package. J Stat Softw 36:1–48
Wood SA, Bowman M (2021) Large-scale farmer-led experiment demonstrates positive impact of cover crops on multiple soil health indicators. Nature Food 2(2):97–103
Download references
Acknowledgements
We would like to acknowledge the contributions of S. Williams to the DayCent model improvements as well as our project Advisory Board members.
This work was supported by United States Department of Agriculture National Institute for Food and Agriculture Organic Program award number 2016-51106-25712 to MES and a NERC Doctoral Training Partnership grant (NE/S007407/1) to HH.
Author information
Authors and affiliations.
Department of Soil and Crop Sciences, Colorado State University, Fort Collins, USA
Meagan E. Schipanski & Keith Paustian
Soil and Crop Sciences, School of Integrative Plant Science, Cornell University, Ithaca, USA
Shelby C. McClelland
Global Academy of Agriculture and Food Systems, University of Edinburgh, Edinburgh, UK
Helen M. Hughes
Department of Plant Sciences, University of Wyoming, Laramie, USA
Randa Jabbour & Jonathan Hillier
Sustainable Food Lab, Burlington, VT, USA
Daniella Malin & Elizabeth Reaves
You can also search for this author in PubMed Google Scholar
Contributions
M.E.S wrote the main manuscript with input from S.M, H.H, and D.M, which represents a synthesis of work contributed by the other authors as cited throughout the manuscript. E.R. coordinated stakeholder surveys. All authors reviewed the manuscript.
Corresponding author
Correspondence to Meagan E. Schipanski .
Ethics declarations
Competing interests.
D. Malin and H. Hughes are involved in the continued development of the Cool Farm Tool and participate in the Cool Farm Alliance. The Cool Farm Alliance is a partner in H. Hughes’ PhD studentship.K. Paustian led development of COMET-Farm.
Additional information
Publisher's note.
Springer Nature remains neutral with regard to jurisdictional claims in published maps and institutional affiliations.
Rights and permissions
Open Access This article is licensed under a Creative Commons Attribution 4.0 International License, which permits use, sharing, adaptation, distribution and reproduction in any medium or format, as long as you give appropriate credit to the original author(s) and the source, provide a link to the Creative Commons licence, and indicate if changes were made. The images or other third party material in this article are included in the article's Creative Commons licence, unless indicated otherwise in a credit line to the material. If material is not included in the article's Creative Commons licence and your intended use is not permitted by statutory regulation or exceeds the permitted use, you will need to obtain permission directly from the copyright holder. To view a copy of this licence, visit http://creativecommons.org/licenses/by/4.0/ .
Reprints and permissions
About this article
Schipanski, M.E., McClelland, S.C., Hughes, H.M. et al. Improving Decision Support Tools for Quantifying GHG Emissions from Organic Production Systems. Org. Agr. (2024). https://doi.org/10.1007/s13165-024-00466-5
Download citation
Received : 07 November 2023
Accepted : 16 April 2024
Published : 26 April 2024
DOI : https://doi.org/10.1007/s13165-024-00466-5
Warm spring weather benefitted crops in most of Europe
According to the April edition of the JRC MARS Bulletin crop monitoring in Europe , most parts of Europe experienced exceptionally warm spring temperatures between early March and mid April.
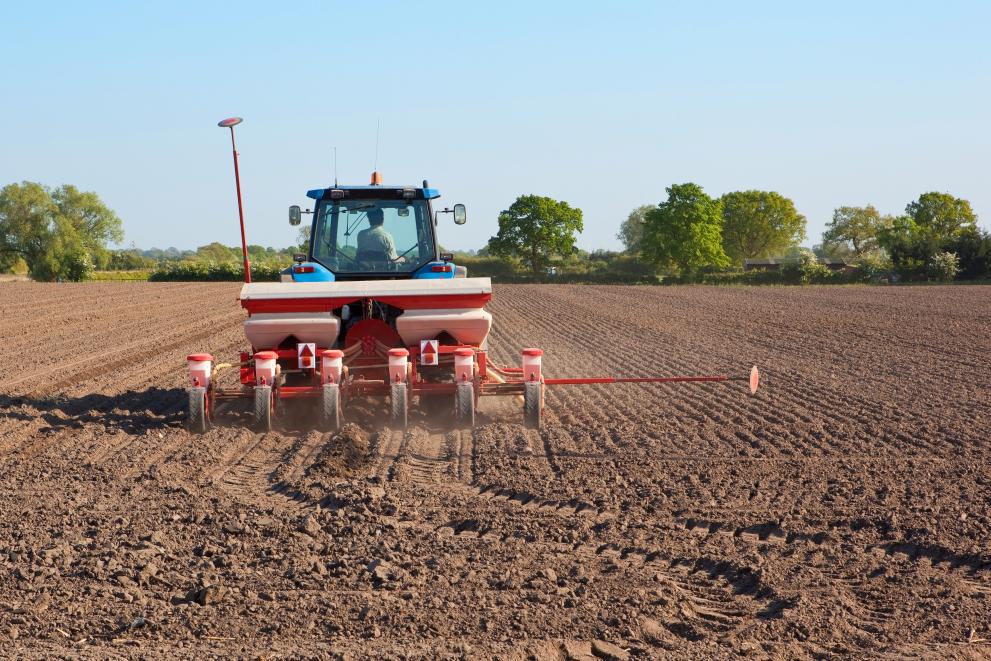
These exceptionally warm spring temperatures, combined with adequate water supply, benefitted winter crops and created favourable conditions for the sowing and emergence of spring cereals and summer crops.
Positive yield outlook for the Iberian Peninsula
Weather conditions have been particularly favourable in the Iberian Peninsula, leading to an upward revision of the yield forecast at EU level for durum wheat, spring barley and triticale.
Negative impacts of persistently wet conditions in north-western Europe
However, overly wet conditions in north-western Europe negatively affected the yield potential and hampered sowing; most severely in Ireland and the United Kingdom. Conditions improved somewhat in northern and part of western France, as well as in Belgium, the Netherlands and north-western Germany, but winter crops in inadequately drained fields are unlikely to fully recover from the overly wet conditions during autumn and winter.
Dry conditions negatively impacted the yield potential in some southern regions
Water deficit have been negatively affecting the development of winter crops in Central Greece and Cyprus.
In Sicilia, as well as in eastern Romania rainfall arrived too late or was too little to fully recover the water stressed winter crops.
Severe irreversible drought impact to winter crops is observed in large parts of Morocco and western Algeria.
New features in the edition of the Bulletin
This edition of the JRC MARS Bulletin contains several new features, such as a new approach for the so-called areas-of-concern analysis (on page 2 and 3); a new style and format of the yield forecast maps with a more nuanced legend and bar and pie diagrams, which allow a better understanding of impacts at country level in an EU perspective; and an additional column in all yield forecast tables that gives the change in the forecast compared with the preceding month.
Further information
JRC MARS (Monitoring Agricultural Resources) Bulletins
JRC AGRI4CAST Toolbox
The latest information about global agricultural production hotspots for countries at risk of food insecurity is available on the JRC’s ASAP (Anomaly hot Spots of Agricultural Production) .
- Sustainable food systems
More news on a similar topic
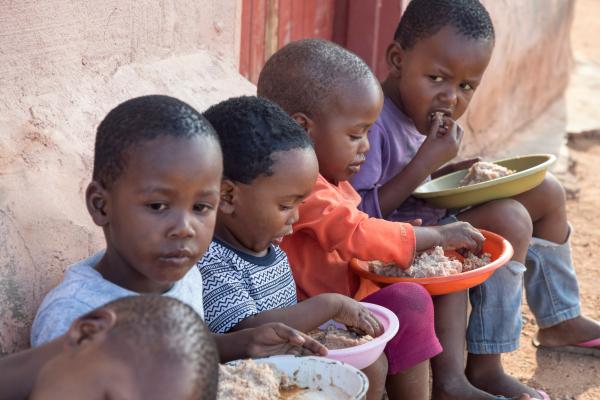
- News announcement
- 24 April 2024
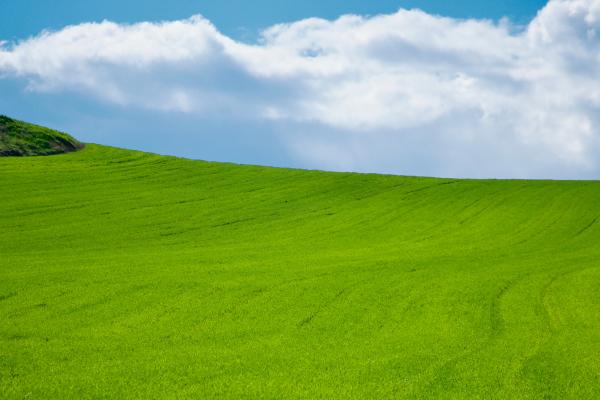
- General publications
- 22 April 2024
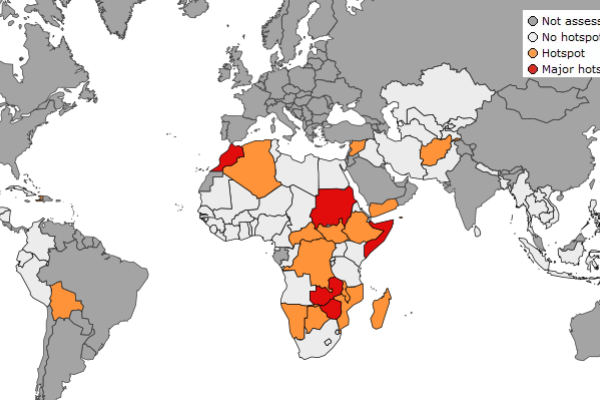
- 12 April 2024
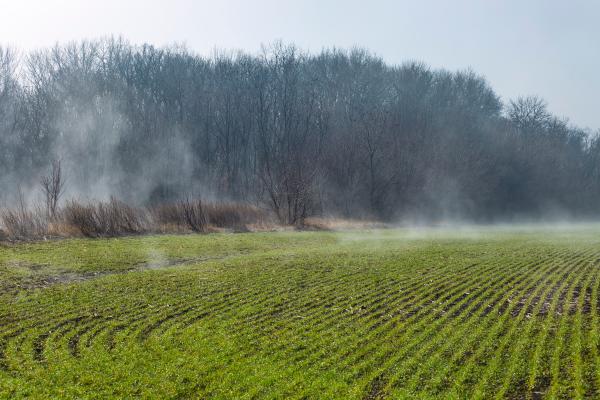
- 25 March 2024
Share this page
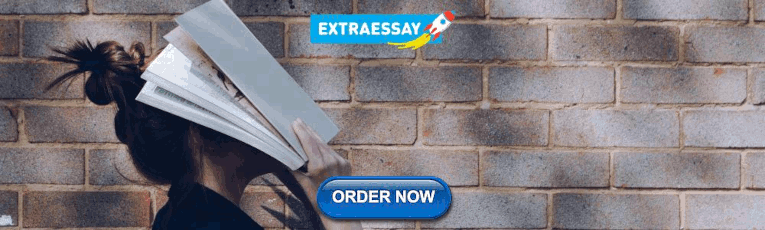
COMMENTS
PDF | On Jan 1, 2015, Abebe Kirub published Agricultural Research Proposal Writing: Addressing Familiar Questions | Find, read and cite all the research you need on ResearchGate
A good full proposal will have a sound, clear and logically linked methodology of implementation and management. The full project proposal should contain no more than fifteen (15) pages of text (Appendixes, table of contents and cover sheets excluded). The number of pages allocated to each section is a guide.
Each Proposal must be supported by one investment Supervising Entity (AfDB, ADB, IFAD, IDB, or WB). In addition, a country may choose to ... agricultural production are marginal or landless farmers; around 60 percent of the employed women were engaged in ... which research closely links to poor crop diversification, poverty
When submitting the full project proposal, additional attachments (endorsement letters, funding commitments, certification of the status of the organization) can also be submitted with the main proposal. Please ensure that the project proposal and all attachments are legible in Times New Roman 12 and provided in two formats (pdf and word).
The study examined socioeconomic determinants of African yam bean production among smallholder crop farmers in Ekiti State. Using a structured questionnaire and interview schedule, data were ...
Enhancing Crop Agronomy has proven to be a crucial aspect of crop improvement in agricultural research for development. By optimizing the management practices and environmental conditions under which crops are grown, researchers aim to maximize yield, enhance resilience against biotic and abiotic stresses, and promote sustainability in agriculture.
agricultural systems and production of different crops. The existence of this diverse agro-ecology together with diverse farming systems, socio-economic, cultures and climate zones provided Ethiopia with various biological wealth of plants, animals, and microbial species, especially crop diversity (Atnaf et al., 2015).
- 3 Attempts to develop one subsector results in imbalances. An example is an emphasis in cash crop production during the colonial periods resulted in neglect of food crops and livestock. A combined approach would have averted imbalances. In addition to this, one finds that even within one crop there is a need to combine several disciplines. If one considers a
1. Introduction. Quantifying the impacts of plant pests and diseases on crop performances represents one of the most important research questions for agricultural simulation modelling (Newman et al., 2003, Savary et al., 2006, Esker et al., 2012, Whish et al., 2015a).In the past, theoretical frameworks were thus developed to take into account the impact of pests and disease on yield as ...
Census of Agriculture (2000 and 2010), the Multiple Frame methodology (FAO 1996 and 1998), working documents on crop forecasting, enumeration of nomadic livestock, estimation of root crop production etc. However, with the decline in attention and priority given to the agriculture sector on the development agenda
A THESIS PROPOSAL SUBMITTED TO THE DEPARTMENT OF AGRO FORESTRY, ... change will reduce agricultural crop productivity in Ethiopia by 5 -10 percent by 2030. Several studies indicate that agriculture production could be significantly impacted due to increase in temperature (Aggarwal et al., 2009; Lobell et al., 2012), changes in rainfall ...
Use this text field to describe the property thoroughly, including topography, soil composition, climate, and recent property improvements. III. Agriculture Proposal Details. This section of the proposal template describes the agriculture project you are developing this proposal for. Select a purpose for the project, then insert a detailed ...
Introduction. Asia is the most populous subcontinent in the world (UNO, 2015), comprising 4.5 billion people—about 60% of the total world population.Almost 70% of the total population lives in rural areas and 75% of the rural population are poor and most at risk due to climate change, particularly in arid and semi-arid regions (Yadav and Lal, 2018; Population of Asia, 2019).
Academia.edu is a platform for academics to share research papers. PROJECT PROPOSAL FOR FOOD CROP PRODUCTION AND SMALL RUMINANTS PREPARED BY: ALISA WOMEN'S FARMING PROJECT FOR FUNDING ... To create a sense of awareness for the women folk towards sustainable agriculture production. To re-organize and strengthen the existing 5 villages.
Tip 4: Timeline. The project timetable would include the project's estimated start and conclusion dates, as well as its length. Giving a schedule would not only assist set expectations but would also make sample budget management easier. Because the cost of production and early financing would have to be considered.
Contents Comparative Efficacy of Pyrethroid Insecticides Against Symphylans in Tall Fescue Seed Crops Industry Survey of Current Practices and Perspectives on Clover Seed Weevil Management in White Clover Seed Production Effect of Spring Defoliation and Trinexapac-ethyl (Palisade EC) Plant Growth Regulator on Annual Ryegrass Seed Crops Soil Carbon Stock Response to Subsurface Drainage in the ...
Vanuatu, 2012. PROPOSAL NARRATIVE. Problem Statement, Research Questions, and Research Objectives. More than 1000 varieties of root and tuber crops are grown across a sample group of 10 villages in the island nation of Vanuatu in the South Pacific. Maintaining this diversity is the foundation of the island inhabitants' food security strategy ...
But it can all be overcome by the large production of agricultural products, this will result in prosperity can be met. ... BUSINESS AND ECONOMICS DEPARTEMENT OF ECONOMICS CROP PRODUCTION AND ITS CONTRIBUTION TO ECONOMIC DEVELOPMENT A RESEARCH PROPOSAL SUBMITTED TO THE DEPARTMENT OF ECONOMICS IN PARTIAL FULFILMENT FOR THE REQUIREMENT OF ...
Problem definition. Cassava (Manihot esculenta), also called yuca or manioc, is extensively cultivated as an annual crop in tropical and subtropical regions for its edible starchy tuberous root, a major source of carbohydrates. Cassava is the third largest source of food carbohydrates in the world.
Abstract. Agricultural robotics is an emerging field that utilizes advanced technologies to revolutionize traditional agricultural practices, enhance crop production efficiency, and contribute to ...
Agriculture has traditionally operated as a circular economic system, utilizing livestock farming yields waste as manure, animal bedding, and related by-products and crop residue 1 to restore soil ...
of the field crop seed and fodder production forages were lost. This is severely threatening the availability of seeds for the coming agricultural seasons, at a time when the Palestinian farmers are already suffering from high prices of agricultural inputs, especially the seeds of field crops and fodder to feed livestock.
The Cool Farm Tool works by providing users with the ability to enter soil and climate characteristics along with agricultural production practices associated with a given crop or food product. For example, the Cool Farm Tool was used by Costco to quantify GHG emissions from their entire supply of domestic organic eggs from 2010 to 2012 (Vetter ...
the designing of crop p roduction agricultural technologies i s proposed. In the d esign process, the following basic principles must be maintained: a syste matic approach, focus on ob taining ...
1. Introduction. One-third of the 800 million people who suffered from chronic malnutrition worldwide in 2017 were found in Africa. Citation 1 Despite having 25% of all arable land, 10% of global agricultural production originates in Africa. Citation 2 Low agricultural productivity can be attributed to several factors, including food loss and spoiling caused by pests and pathogenic microorganisms.
Agrivoltaics is the combination of solar panels and agricultural production at the same location. Traditionally agrivoltaics referred to systems with crops—typically fruits or vegetables—grown under solar panels, but the term has evolved to include combining solar panels with grazing livestock (mainly sheep) and planting native grasses or pollinator habitat beneath solar panels.
JRC MARS (Monitoring Agricultural Resources) Bulletins. JRC AGRI4CAST Toolbox. The latest information about global agricultural production hotspots for countries at risk of food insecurity is available on the JRC's ASAP (Anomaly hot Spots of Agricultural Production).
Agriculture plays prominent role in the process of economic development for a country; without achieving substantial increase in agricultural production, no country has moved to take off stage of ...