The Library wishes you a nice holiday break. Buildings will be closed from 12/23/22 to 12/31/22. For a full list of closing and opening times, please visit the library hours page.
- Undergraduate Students
- Graduate & Medical Students
- Medical & Clinical Faculty
- Visiting Scholars
- Special Collections Researchers
- Library Staff
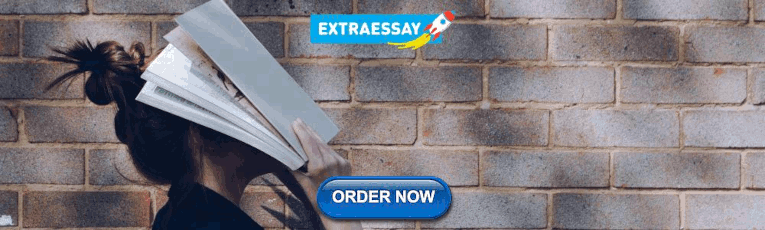
Brown University Theses and Dissertations
Brown University Library archives dissertations in accordance with the Brown Graduate School policy .
For dissertations published prior to 2008, please consult the following Dissertation LibGuide
Active filters
Refine your results.
- 5 Biology and Medicine
- 5 Biomedical Engineering
- 4 Chemistry
- 1 Computer Science
- 3 Engineering
- Show More...
- 2 Bachelors Thesis
- 14 Doctoral Dissertation
- 1 Aluminum alloys
- 1 Aluminum oxide
- 1 Aluminum--Anodic oxidation
- 1 Antibacterial agents
- 1 Fluid, Thermal, and Chemical Processes
- 1 Mechanics of Solids
- 1 Artificial intelligence
- 1 Bio-sensors
- 1 Biodegradation
- 1 Bioengineering
- 1 Biomedical engineering
- 1 Biomedical engineering--Research
- 1 Biomimetics
- 1 Biosensing
- 2 Biosensors
- 1 Biotechnology
- 1 Blood-vessels
- 1 Bone Implants
- 1 Cancer cells
- 1 Cell functions
- 1 Chemical engineering
- 1 Coded Computation
- 1 Computational science and engineering
- 1 Contrast media (Diagnostic imaging)
- 1 Deep learning (Machine learning)
- 1 Defective Graphene
- 1 Detection
- 1 Diagnosis
- 1 Diagnostics
- 1 Drug Delivery
- 1 Drug delivery systems
- 1 Drug-delivery
- 1 Electrokinetics
- 1 Emerging Nanomaterials
- 1 Engineering
- 1 Engineering design
- 1 Environment
- 1 Environmental degradation
- 1 Fabry-Perot interferometers
- 1 Fault-Tolerance
- 1 Functionalization
- 1 Gadolinium
- 1 Health risk assessment
- 1 Integrated circuits--Fault tolerance
- 1 Ion Batteries
- 1 Magnetic Nanoparticles
- 1 Magnetic resonance imaging
- 1 Material Science
- 1 Materials science
- 1 Medical instruments and apparatus
- 1 Microelectromechanical systems
- 1 Microelectronics
- 1 Microfluidic devices
- 1 Microfluidics
- 1 Molecular diagnosis
- 1 Molybdenum disulfide
- 1 Nano-Selenium
- 2 Nanomaterials
- 1 Nanomechanics
- 1 Nanomedicine
- 1 Nanoparticle Characterization
- 1 Nanoparticles
- 1 Nanopores
- 1 Nanoscience
- 3 Nanostructured materials
- 16 Nanotechnology
- 1 Nanowire Decoders
- 1 Nanowires
- 1 Orthopedic implants
- 1 Power resources
- 1 Probabilistic Analysis
- 1 Radiographic contrast media
- 1 Remediation
- 1 Self-assembly (Chemistry)
- 1 Single molecular test tube
- 1 Solid Mechanics
- 1 Stochastic Assembly
- 1 Surface chemistry
- 1 Surface properties
- 1 Targeting
- 1 Theranostics
- 1 Thin films--Optical properties
- 1 Toxicology
- 1 Vascular stents
- 1 anodic alumina
- 1 anodization
- 1 anodized aluminum
- 1 antibacterial
- 1 antibiotic resistance
- 1 biomaterial
- 1 bone tissue engineering
- 1 cancer screening
- 1 coloration
- 1 data science
- 1 diagnostic test
- 1 diagnostics
- 1 fabry perot
- 1 filamentation
- 1 metabolic simulation
- 1 nanocrystalline hydroxyapatite
- 2 nanofabrication
- 1 nanomaterials
- 1 nanorough surface
- 1 optical cavity
- 1 optical rectification
- 1 optical resonator
- 1 physical coloration
- 1 resistive switching
- 1 rosette nanotubes
- 1 self-assembly
- 1 structural coloration
- 1 superparamagnetic iron oxide nanoparticles (SPION)
- 1 tissue engineering
Items (1-16) out of 16 results
- title (A-Z)
- title (Z-A)
- date (old to new)
- date (new to old)
- new to the BDR
- 10 per page
- 20 per page
- 50 per page
- 100 per page
Anodic alumina as a scalable platform for structural coloration and optical rectification
Biological Application of Magnetic Nanoparticles in Targeted Therapeutics and Diagnostics
Biologically Inspired Rosette Nanotube Nanocomposites for Bone Tissue Engineering, Orthopedic and Vascular Applications
Biologically Relevant Degradation of 2D Nanomaterials: Kinetics, Hazard Classification and Biomedical Applications
Enhanced Efficacy of Nanotechnology-Driven Approaches against Antibiotic-Resistant Biofilms in the Presence of Metabolites
Evaluating the Human Health and Environmental Impacts of Exposure to Two-Dimensional Manganese Dioxide Nanosheets
Nano-fabrication and Characterization of Novel Titanium Surfaces for Vascular Stent Application
Nano-Selenium: Novel Formulations for Biological and Environmental Applications
Nanopatterned PLGA for Anti-cancer Implant Applications
Novel Devices, Physical Mechanisms, and Analytical Techniques for Use in Next Generation Cellular Diagnostics
Novel Polymers as Phase Transfer Agents for Gadolinium Oxide Nanoplates: Improving Magnetic Resonance Imaging Contrast
Reliable Computing at the Nanoscale
Select Nanofabricated Titanium Materials for Enhancing Bone and Skin Growth of Intraosseous Transcutaneous Amputation Prostheses
Structural and optical characterization of diamond nanowires
The Use of Entropic Cages For Trapping DNA and Controlling its Configurations in Nanopore Studies
Topics in Nanomechanics, Energy Storage Systems, and Emerging Nanomaterials
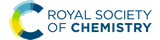
Nanomaterials: a review of synthesis methods, properties, recent progress, and challenges

First published on 24th February 2021
Nanomaterials have emerged as an amazing class of materials that consists of a broad spectrum of examples with at least one dimension in the range of 1 to 100 nm. Exceptionally high surface areas can be achieved through the rational design of nanomaterials. Nanomaterials can be produced with outstanding magnetic, electrical, optical, mechanical, and catalytic properties that are substantially different from their bulk counterparts. The nanomaterial properties can be tuned as desired via precisely controlling the size, shape, synthesis conditions, and appropriate functionalization. This review discusses a brief history of nanomaterials and their use throughout history to trigger advances in nanotechnology development. In particular, we describe and define various terms relating to nanomaterials. Various nanomaterial synthesis methods, including top-down and bottom-up approaches, are discussed. The unique features of nanomaterials are highlighted throughout the review. This review describes advances in nanomaterials, specifically fullerenes, carbon nanotubes, graphene, carbon quantum dots, nanodiamonds, carbon nanohorns, nanoporous materials, core–shell nanoparticles, silicene, antimonene, MXenes, 2D MOF nanosheets, boron nitride nanosheets, layered double hydroxides, and metal-based nanomaterials. Finally, we conclude by discussing challenges and future perspectives relating to nanomaterials.
1. Introduction
The term nanometer was first used in 1914 by Richard Adolf Zsigmondy. 5 The American physicist and Nobel Prize laureate Richard Feynman introduced the specific concept of nanotechnology in 1959 in his speech during the American Physical Society's annual meeting. This is considered to be the first academic talk about nanotechnology. 5 He presented a lecture that was entitled “There's Plenty of Room at the Bottom”. During this meeting, the following concept was presented: “why can’t we write the entire 24 volumes of the Encyclopedia Britannica on the head of a pin?” The vision was to develop smaller machines, down to the molecular level. 6,7 In this talk, Feynman explained that the laws of nature do not limit our ability to work at the atomic and molecular levels, but rather it is a lack of appropriate equipment and techniques that limit this. 8 Through this, the concept of modern technology was seeded. Due to this, he is often considered the father of modern nanotechnology. Norio Taniguchi might be the first person who used the term nanotechnology, in 1974. Norio Taniguchi stated: “nano-technology mainly consists of the processing of, separation, consolidation, and deformation of materials by one atom or one molecule.” 5,9 Before the 1980s, nanotechnology remained only an area for discussion, but the concept of nanotechnology was seeded in the minds of researchers with the potential for future development.
The invention of various spectroscopic techniques sped up research and innovations in the field of nanotechnology. IBM researchers developed scanning tunneling microscopy (STM) in 1982, and with STM it became feasible to attain images of single atoms on “flat” ( i.e. , not a tip) surfaces. 10 Atomic force microscopy (AFM) was invented in 1986, and it has become the most crucial scanning probe microscope technique. 11 The motivation to develop hard discs with high storage density stimulated the measurement of electrostatic and magnetic forces. This led to the development of Kelvin-probe-, electrostatic-, and magnetic-force microscopy. 12 Currently, nanotechnology is rapidly evolving and becoming part of almost every field related to materials chemistry. The field of nanotechnology is evolving every day, and now powerful characterization and synthesis tools are available for producing nanomaterials with better-controlled dimensions.
Nanotechnology is an excellent example of an emerging technology, offering engineered nanomaterials with the great potential for producing products with substantially improved performances. 13 Currently, nanomaterials find commercial roles in scratch-free paints, surface coatings, electronics, cosmetics, environmental remediation, sports equipment, sensors, and energy-storage devices. 14 This review attempts to provide information in a single platform about the basic concepts, advances, and trends relating to nanomaterials via covering the related information and discussing synthesis methods, properties, and possible opportunities relating to the broad and fascinating area of nanomaterials ( Scheme 1 ). It is not easy to cover all the literature related to nanomaterials, but important papers from history and the current literature are discussed in this review. This review provides fundamental insight for researchers, quickly capturing the advances in and properties of various classes of nanomaterials in one place.
2. Descriptions of terms associated with nanomaterials
3. approaches for the synthesis of nanomaterials, 3.1. top-down approaches.
The conditions under which arc discharge takes place play a significant role in achieving new forms of nanomaterials. The conditions under which different carbon-based nanomaterials are formed via the arc discharge method are explained in Fig. 6 . Various carbon-based nanomaterials are collected from different positions during the arc discharge method, as their growth mechanisms differ. 44 MWCNTs, high-purity polyhedral graphite particles, pyrolytic graphite, and nano-graphite particles can be collected from either anode or cathode deposits or deposits at both electrodes. 46–48 Apart from the electrodes, carbon-based nanomaterials can also be collected from the inner chamber. Different morphologies of single-wall carbon nanohorns (SWCNHs) can be obtained under different atmospheres. For example, ‘dahlia-like’ SWCNHs are produced under an ambient atmosphere, whereas ‘bud-like’ SWCNHs are generated under CO and CO 2 atmospheres. 49 The arc discharge method can be used to efficiently achieve graphene nanostructures. The conditions present during the synthesis of graphene can affect its properties. Graphene sheets prepared via a hydrogen arc discharge exfoliation method are found to be superior in terms of electrical conductivity and have good thermal stability compared to those obtained via argon arc discharge. 50
3.2. Bottom-up approaches
The hard template method is also called nano-casting. Well-designed solid materials are used as templates, and the solid template pores are filled with precursor molecules to achieve nanostructures for required applications ( Fig. 10 ). 78 The selection of the hard template is critical for developing well-ordered mesoporous materials. It is desirable that such hard templates should maintain a mesoporous structure during the precursor conversion process, and they should be easily removable without disrupting the produced nanostructure. A range of materials has been used as hard templates, not limited to carbon black, silica, carbon nanotubes, particles, colloidal crystals, and wood shells. 85 Three main steps are involved in the synthetic pathway for obtaining nanostructures via templating methods. In the first step, the appropriate original template is developed or selected. Then, a targeted precursor is filled into the template mesopores to convert them into an inorganic solid. In the final step, the original template is removed to achieve the mesoporous replica. 86 Via using mesoporous templates, unique nanostructured materials such as nanowires, nanorods, 3D nanostructured materials, nanostructured metal oxides, and many other nanoparticles can be produced. 87 From this brief discussion, it can be seen that a wide range of unique structured nanomaterials can be produced using soft and hard template methods.
4. Unique nanomaterial features
The electronic properties of semiconductors in the 1–10 nm range are controlled by quantum mechanical considerations. Thus, nanospheres with diameters in the range of 1–10 nm are known as quantum dots. The optical properties of nanomaterials such as quantum dots strongly depend upon their shape and size. 96 A photogenerated electron–hole pair has an exciton diameter on the scale of 1–10 nm. Thus, the absorption and emission of light by semiconductors could be controlled via tuning the nanoparticle size in this range. However, in the case of metals, the mean free path of electrons is ∼10–100 nm and, due to this, electronic and optical effects are expected to be observed in the range of ∼10–100 nm. The colors of aqueous solutions of metal nanoparticles can be changed via changing the aspect ratio. Aqueous solutions of Ag NPs show different colors at different aspect ratios. A red shift in the absorption band appears with an increase in the aspect ratio ( Fig. 12 ). 21
Among a range of unique properties, the following key properties can be obtained upon tuning the sizes and morphologies of nanomaterials.
4.1. Surface area
4.2. magnetism, 4.3. quantum effects, 4.4. high thermal and electrical conductivity, 4.5. excellent mechanical properties, 4.6. excellent support for catalysts, 4.7. antimicrobial activity.
Overall, these features have made nanoscale materials valuable for a wide range of applications, substantially boosting the performances of various devices and materials in a number of fields. Details of various nanomaterials, their properties, and applications in various fields will be discussed below.
5. Nanomaterials, characteristics, and applications
5.1. special carbon-based nanomaterials.
In the carbon-based nanomaterial family, fullerenes were the first symmetric material, and they provided new perspectives in the nanomaterials field. This led to the discovery of other carbon-based nanostructured materials, such as carbon nanotubes and graphene. 110 Fullerenes are present in nature and interstellar space. 111 Interestingly, fullerenes were the molecule of the year in 1991 and attracted the most research projects compared to other scientific subjects during that period. 112 Fullerenes possess several unique features that make them attractive for applications in different fields. Fullerenes display solubility to some extent in a range of solvents, and these characteristics make them unique compared to the other allotropes of carbon. 108
The chemical modification of fullerenes is an exciting subject, improving their effectiveness for applications. There are two main ways to modify fullerenes: 113 fullerene inner-space modification, and fullerene outer-surface modification.
Endohedral and exohedral doping examples are shown in Fig. 13 . 114 Fullerenes are hollow cages, and the interior acts as a robust nano-container for host target species when forming endohedral fullerene. 115 Endohedral fullerenes do not always follow the isolated pentagon rule (IPR). 116 To date, fullerene nanocages have received substantial consideration in the materials chemistry field due to their useful potential applications. Neutral and charged single atoms in free space are highly reactive and unstable. In the confined environment of fullerenes, these reactive species can be stabilized; for example, the LaC 60 + ion does not react with the NH 3 , O 2 , H 2 , or NO. Thus, reactive metals can be protected from the surrounding environment by trapping them inside fullerene cages. 117 Another emerging carbon nanomaterial is endohedral fullerene containing lithium (Li@C 60 ). 118 Lithium metal is very reactive, and extreme controlled environmental conditions are required to preserve or use it. In other words, secure structures are required for lithium storage. Li-Based endohedral fullerene shows unique solid-state properties. The encapsulation of lithium atoms in fullerene helps to protect lithium atoms from external agents. Li-Based endohedral fullerenes have the potential to open the door to nanoscale lithium batteries. 119 For the development of endohedral metallofullerenes, larger fullerenes are generally required, as they possess large cages to accommodate lanthanide and transition metal atoms more smoothly. 118 Fullerene nanocages are useful for the storage of gases. Fullerene is under consideration for hydrogen storage. 120,121
Exohedral fullerenes carry more potential for applications as outer surfaces can be more easily modified or functionalized. The exohedral doping of metals into fullerenes strongly affects the electronic properties via shifting electrons from the metal to the fullerene nanocage. 122 The practical application of fullerenes can be achieved with tailor-made fullerene derivatives via chemical functionalization. As fullerene chemistry has matured, a wide range of functionalized fullerenes has been realized through simple synthetic routes. 123 The combination of hydrogen-bonding motifs and fullerenes has allowed the modulation of 1D, 2D, and 3D fullerene-based architectures. 124 The excellent electron affinities of fullerenes have shown great potential for eliminating reactive oxygen species. The presence of excess reactive oxygen species can cause biological dysfunction or other health issues. The surfaces of fullerenes have been functionalized via mussel-inspired chemistry and Michael addition reactions for the fabrication of C 60 –PDA–GSH. The developed C 60 –PDA–GSH nanoparticles demonstrated excellent potential for scavenging reactive oxygen species. 125
Amphiphiles have great importance in industrial processes and daily life applications. Amphiphilic molecules consist of hydrophilic and hydrophobic parts, and they perform functions in water via forming two- and three-dimensional assemblies. Recently, conical fullerene amphiphiles 126 have emerged as a new class of amphiphiles, in which a nonpolar apex is supplied by fullerenes and a hydrophilic part is achieved through functionalization. The selective functionalization of the fullerene on one side helps to achieve a supramolecule due to unique interfacial behavior. The unique supramolecular structure formed via the spontaneous assembly of one-sided selectively functionalized fullerenes through strong hydrophobic interactions between the fullerene apexes and polar functionalized portions is soluble in water. Conical fullerene amphiphiles are mechanically robust. Via upholding the structural integrity, conical fullerene amphiphiles can be readily aggregated with nanomaterials and biomolecules to form multicomponent agglomerates with controllable structural features. 127 Fullerenes, after suitable surface modification, have excellent potential for use in drug delivery, but there have only been limited explorations of their drug delivery applications. 128,129 Fullerene-based nano-vesicles have been developed for the delayed release of drugs. 130 Water-soluble proteins have great potential in the field of nanomedicine. The water-soluble cationic fullerene, tetra(piperazino)[60] fullerene epoxide (TPFE), has been used for the targeted delivery of DNA and siRNA specifically to the lungs. 131 For diseases in lungs or any other organ, efficient treatment requires the targeted delivery of active agents to a targeted place in the organ. The accumulation of micrometer-sized carriers in the lung makes lung-selective delivery difficult, as this may induce embolization and inflammation in the lungs. Size-controlled blood vessel carrier vehicles have been developed using tetra(piperazino)fullerene epoxide (TPFE). TPFE and siRNA agglutinate in the bloodstream with plasma proteins and, as a result, micrometer-sized particles are formed. These particles clog the lung capillaries and release siRNA into lungs cells; after siRNA delivery, they are immediately cleared from the lungs ( Fig. 14 ). 132
The supramolecular organization of fullerene (C 60 ) is a unique approach for producing shape-controlled moieties on the nano-, micro-, and macro-scale. Nano-, micro-, and macro-scale supramolecular assemblies can be controlled via manipulating the preparation conditions to achieve unique optoelectronic properties. 133 The development of well-ordered and organized 1D, 2D, and 3D fullerene assemblies is essential for achieving advanced optical and organic-based electronic devices. 134 Fullerene-based nanostructured materials with new dimensions are being developed from zero-dimensional fullerene and tuned to achieve the desired characteristics. 1D C 60 fullerene nanowires have received substantial attention over other crystalline forms due to their excellent features of potential quantum confinement effects, low dimensionality, and large surface areas. 135
Carbon nanomaterials are also used as supports for catalysts, and the main reasons to use them are their high surface areas and electrical conductivities. Carbon supports strongly influence the properties of metal nanoparticles. In fuel cells, the carbon support strongly affects the stability, electronic conductivity, mass transport properties, and electroactive surface area of the supported catalyst. 136 In fuel cells, the degradation of some catalysts, such as platinum-based examples, and carbon is correlated and reinforced as a result of both being present. Carbon support oxidation is catalyzed by platinum and the oxidation of carbon accelerates platinum-catalyst release. Overall, this results in a loss of catalytically active surface area. 137 Fullerenes are considered suitable support materials due to their excellent electrochemical activities and stability during electrochemical reactions. 138 Due to their high stability and good conductivity, fullerenes can replace conventional carbon as catalyst support materials. Fullerenes are also used for the development of efficient solar cells. 139
Apart from the applications mentioned above, fullerenes have a broader spectrum of applications where they can be used to improve outcomes considerably. Fullerenes have the potential to be used in the development of superconductors. 140 The strong covalent bonds in fullerenes make them useful nanomaterials for improving the mechanical properties of composites. 141 The combination of fullerenes with polymers can result in good flame-retardant and thermal properties. 142 Fullerenes and their derivatives are used for the development of advanced lubricants. They are used as modifiers for greases and individual solid lubricants. 138 Fullerenes have tremendous medicinal importance due to their anticancer, antioxidant, anti-bacterial, and anti-viral activities. 104
Fullerenes are vital members of the carbon-based nanomaterial family and they certainly possess exceptional properties. This discussion further emphasizes their importance for advanced applications. However, the discovery of other carbon-based nanomaterials has put fullerenes in the shade, and the pace of their exploration has been reduced. As fullerenes are highly symmetrical molecules with unique properties, they can act as performance boosters, but more attention is needed from researchers for their practical expansion. 110
Single-walled carbon nanotubes consist of a seamless one-atom-thick graphitic layer, in which carbon atoms are connected through strong covalent bonds. 146 Double-walled carbon nanotubes consist of two single-walled carbon nanotubes. One carbon nanotube is nested in another nanotube to construct a double-walled carbon nanotube. 147 In multi-walled carbon nanotubes, multiple sheets of single-layer carbon atom are rolled up. In other words, many single-walled carbon nanotubes are nested within each other. From different types of nanotubes, it can be concluded that the nanotubes may consist of one, tens, or hundreds of concentric carbon shells, and these shells are separated from each other with a distance of ∼0.34 nm. 148 Carbon nanotubes can be synthesized via chemical vapor deposition, 149 laser ablation, 150 arc-discharge, 143 and gas-phase catalytic growth. 151
Single-walled carbon nanotubes display a diameter of 0.4 to 2 nm. The inner wall distance between double-walled carbon nanotubes was found to be in the range of 0.33 to 0.42 nm. MWCNT diameters are usually in the range of 2–100 nm, and the inner wall distance is about 0.34 nm. 147,152 However, it is essential to note that the diameters and lengths of carbon nanotubes are not well defined, and they depend on the synthesis route and many other factors. The electrical conductivities of SWCNTs and MWCNTs are about 10 2 –10 6 S cm −1 and 10 3 –10 5 S cm −1 , respectively. SWCNTs and MWCNTs also display excellent thermal conductivities of ∼6000 W m −1 K −1 and ∼2000 W m −1 K −1 , respectively. CNTs remain stable in air at temperatures higher than 600 °C. 153 These properties indicate that CNTs have obvious advantages over graphite.
Single-walled carbon nanotubes can display metallic or semiconducting behavior. Whether carbon nanotubes show metallic or semiconducting behavior depends on the diameter and helicity of the graphitic rings. 154 The rolling of graphene sheets leads to three different types of CNTs: chiral, armchair, and zigzag ( Fig. 15 ). 155
Carbon nanotubes demonstrate some amazing characteristics that make them valuable nanomaterials for possible practical applications. Theoretical and experimental studies of carbon nanotubes have revealed their extraordinary tensile properties. J. R. Xiao et al. used an analytical molecular structural mechanics model to predict SWCNT tensile strengths of 94.5 (zigzag nanotubes) and 126.2 (armchair nanotubes) GPa. 156 In another study, the Young's modulus and average tensile strength of millimeter-long multi-walled carbon nanotubes were analyzed and found to be 34.65 GPa and 0.85 GPa, respectively. 157 Carbon nanotubes possess a high aspect ratio. Due to their high tensile strength, carbon nanotubes are used to enhance the mechanical properties of composites.
Carbon nanotubes have become an important industrial material and hundreds of tonnes are produced for applications. 158 Their high tensile strength and high aspect ratio have made carbon nanotubes an ideal reinforcing agent. 159 Carbon nanotubes are lightweight in nature and are used to produce lightweight and biodegradable nanocomposite foams. 160 The structural parameters of carbon nanotubes define whether they will be semiconducting or metallic in nature. This property of carbon nanotubes is considered to be effective for their use as a central element in the design of electronic devices such as rectifying diodes, 161 single-electron transistors, 162 and field-effect transistors. 163 The chemical stability, nano-size, high electrical conductivity, and amazing structural perfection of carbon nanotubes make them suitable for electron field emitter applications. 164 The unique set of mechanical and electrochemical properties make CNTs a valuable smart candidate for use in lithium-ion batteries. 165 CNTs have the full potential to be used as a binderless free-standing electrode for active lithium-ion storage. CNT-based anodes can have reversible lithium-ion capacities exceeding 1000 mA h g −1 , and this is a substantial improvement compared with conventional graphite anodes. In short, the following factors play a role in controlling and optimizing the performances of CNT-based composites: 166 (i) the volume fraction of carbon nanotubes; (ii) the CNT orientation; (iii) the CNT matrix adhesion; (iv) the CNT aspect ratio; and (iv) the composite homogeneity.
For some applications, a proper stable aqueous dispersion of CNTs at a high concentration is pivotal to allow the system to perform its function efficiently and effectively. 167 One of the major issues associated with carbon nanotubes is their poor dispersion in aqueous media due to their hydrophobic nature. Clusters of CNTs are formed due to van der Waals attraction, π–π stacking, and hydrophobicity. The CNT clusters, due to their strong interactions, hinder solubility or dispersion in water or even organic-solvent-based systems. 168 This challenging dispersion associated with CNTs has limited their use for promising applications, such as in biomedical devices, drug delivery, cell biology, and drug delivery. 167 Carbon nanotube applications and inherent characteristics can be further tuned via suitable functionalization. The functionalization of carbon nanotubes helps scientists to manipulate the properties of carbon nanotubes and, without functionalization, some properties are not attainable. 169 The functionalization of nanotubes can be divided into two main categories: covalent functionalization and non-covalent functionalization.
The heating of CNTs under strongly acidic and oxidative conditions results in the formation of oxygen-containing functionalities. These functional groups, such as carboxylic acid, react further with other functional groups, such as amines or alcohols, to produce amide or ester linkages on the carbon nanotubes. 172 One of the main issues preventing the utilization of CNTs for biomedical applications is their toxicity. The cytotoxicity of pristine carbon nanotubes can be reduced via introducing carbonyl, –COOH, and –OH functional groups. Apart from functionalization through oxidized CNTs, the direct functionalization of CNTs is also possible. However, direct functionalization requires more reactive species to directly react with the CNTs, such as free radicals. Addition reactions to CNTs can cause a transformation from sp 2 hybridization to sp 3 hybridization at the point of addition. At the point where functionalization has taken place, the local bond geometry is changed from trigonal planar to tetrahedral geometry. Some addition reactions to the sidewalls of CNTs are shown in Fig. 16 . 155
It is important to discuss how the covalent functionalization of carbon nanotubes comes at the price of the degradation of the carbon sp 2 network. This substantially affects the electronic, thermal, and optoelectronic properties of the carbon nanotubes. 169 Efforts are being made to introduce a new method of covalent functionalization that can keep the π network of CNTs intact. Antonio Setaro et al. introduced a new [2+1] cycloaddition reaction for the non-destructive, covalent, gram-scale functionalization of single-walled carbon nanotubes. The reaction rebuilds the extended π-network, and the carbon nanotubes retained their outstanding quantum optoelectronic properties ( Fig. 17 ). 173
Polymers are frequently combined with CNTs to enhance their dispersion capabilities. Polymers interact with CNTs through CH–π and π–π interactions. 174 Hexanes and cycloalkanes are poor CNT solvents but the good solubility or dispersion of CNTs in these solvents is required for surface coating applications. Poly(dimethylsiloxane) (PDMS) macromer-grafted polymers have been prepared using PDMS macromers and pyrene-containing monomers that strongly adsorb on CNTs, thus improved the solubility of CNTs in chloroform and hexane. 176 The use of head–tail surfactants is another attractive way to achieve a fine dispersion of CNTs in an aqueous medium. In head–tail surfactants, the tail is hydrophobic and interacts with the CNT sidewalls, and the hydrophilic head groups interact with the aqueous environment to provide a fine dispersion. 177
For electrical applications, non-covalently functionalized CNTs are more preferred because the electrical properties of the CNTs are not compromised. CNTs have been non-covalently functionalized with a variety of biomolecules for the fabrication of electrochemical biosensors. 175 Non-covalently functionalized SWCNTs are used for energy applications. Single-walled carbon nanotubes (SWCNTs) have been non-covalently functionalized with 3d transition metal( II ) phthalocyanines, lowering the potential of the oxygen evolution reaction by approximately 120 mV compared with unmodified SWCNTs. 178 The toxicity of pristine CNTs toward living organisms can be lessened via using surfactant-functionalized CNTs. 170 However, in some cases, during polymer non-covalent functionalization, the polymer may wrap CNT bundles and make it difficult to separate the CNTs from each other. Polymers can develop into insulating wrapping that affects the CNT conductivity.
In the literature, several graphene-related materials have been reported, such as graphene oxide and reduced graphene oxide. 187 Among graphenoids, graphene oxide is a more reported and explored graphene-related material as a precursor for chemically modified graphene. The synthetic route to graphene oxide is straightforward, and it is synthesized from inexpensive graphite powder that is readily available. 188 Graphene oxide has many oxygen-containing functional groups, such as epoxy, hydroxyl, carboxyl, and carbonyl groups. The basal plane of graphene oxide is generally decorated with epoxide and hydroxyl groups, whereas the edges presumably contain carboxyl- and carbonyl-based functional groups. 189 The presence of active functional groups in graphene oxide allows its further functionalization with different polymers, small organic compounds, or other nanomaterials to realize several applications. 190
Graphene oxide, due to its oxygen functionality, is insulating in nature and displays poor electrochemical performance. The presence of oxygen functionalities in graphene oxide breaks the conjugated structure and localizes the π-electron network, resulting in poor carrier mobility and carrier concentration. 196 Its electrochemical performance is improved substantially after removing the oxygen-containing functional groups. 197 These functional groups can be removed or reduced via thermal, electrochemical, and chemical means. The product obtained after removing or reducing oxygen moieties is called reduced graphene oxide. The properties of reduced graphene oxide depend upon the effective removal of oxygen moieties from graphene oxide. The process used to remove oxygen-containing functionalities from graphene oxide will determine the extent to which reduced the properties of graphene oxide resemble pristine graphene. 198
Reduced graphene oxide is extensively used to improve the performances of various electrochemical devices. 199 It is essential to mention that even after reducing graphene oxide, some residual sp 3 carbon bonded to oxygen still exists, which somehow disturbs the movement of charge through the delocalized electronic cloud of the sp 2 carbon network. 200 Apart from this, the electrochemical activity of reduced graphene oxide is substantially high enough to manufacture electrochemical devices with improved performances. Recently, the demand for super-performance electrochemical devices has increased to overcome modern challenges relating to electronics and energy-storage devices. 201 Graphene-based materials are considered to be excellent electrode materials, and they can be proved to be revolutionary for use in energy-storage devices such as supercapacitors (SC) and batteries. Graphene-based electrodes improve the performances of existing batteries (lithium-ion batteries) and they are considered useful for developing next-generation batteries such as sodium-ion batteries, lithium–O 2 batteries, and lithium–sulfur batteries ( Fig. 18 ). Being flat in nature, each carbon atom of graphene is available, and ions can easily access the surface due to low diffusion resistance, which provides high electrochemical activity. 202
Graphene and its derivatives are extensively used for the development of electrochemical sensors. 203 The surfaces of bare electrodes are usually not able to sense analytes at trace levels and they cannot differentiate between analytes that have close electrooxidation properties due to their poor surface kinetics. The addition of graphene layers to the surfaces of electrodes can substantially improve the electrocatalytic activity and surface sensitivity towards analytes. 204 Graphene has definite advantages over other materials that are used as electrode materials for sensor applications. Graphene has a substantially high surface-to-volume ratio and atomic thickness, making it extremely sensitive to any changes in its local environment. This is an essential factor in developing advanced sensing tools, as all the carbon atoms are available to interact with target species.
Consequently, graphene exhibits higher sensitivity than its counterparts such as CNTs and silicon nanowires. 205 Graphene has two main advantages over CNTs for the development of electrochemical sensors. First, graphene is mostly produced from graphite, which is a cost-effective route, and second, graphene does not contain metallic impurities like CNTs can. Graphene offers many other advantages when developing sensors and biosensors, such as biocompatibility and π–π stacking interactions with biomolecules. 206 Graphene-based materials are ideal for the construction of nanostructured sensors and biosensors.
The mechanical properties of graphene are used to fabricate highly desired stretchable and flexible sensors. 207 Graphene can be utilized to develop transparent electrodes with excellent optical transmittance. It displays good piezoresistive sensitivity. Researchers are making efforts to replace conventional brittle indium tin oxide (ITO) electrodes with flexible graphene electrodes in optoelectronic devices such as liquid-crystal displays and organic light-emitting diodes. 208 For human–machine interfaces, transparent and flexible tactile sensors with high sensitivity have become essential. Graphene film (GF) and PET have been applied to develop transparent tactile sensors that exhibit outstanding cycling stability, fast response times, and excellent sensitivity ( Fig. 19 ). 209 Similarly, graphene is applied for the fabrication of pressure sensors. 210 Overall, graphene is an excellent material for developing transparent and flexible devices. 211,212
The use of graphene-based materials is an effective way to deal with a broad spectrum of pollutants. 213 There are many ways to deal with environmental pollution; among these, adsorption is an effective and cost-effective method. 214,215 Graphene-based adsorbents are found to be useful in the removal of organic, 216 inorganic, and gaseous contaminants. Graphene-based materials have some obvious advantages over CNT-based adsorbents. For example, graphene sheets offer two basal planes for contaminant adsorption, enhancing their effectiveness as an adsorbent. 192 GO contains several oxygen functional groups that impart hydrophilic features. Due to appropriate hydrophilicity, GO-based adsorbents can efficiently operate in water to remove contaminants. Moreover, graphene-oxide-based materials can be functionalized further through reactive moieties with various organic molecules to enhance their adsorption capacities. 217
In short, extensive research must continue in order to develop graphene-based materials with high performance and bring them to the market. Massive focus on graphene research is also justified due to the extraordinary features described in extensive theoretical and experimental research works.
Nanodiamonds possess a core–shell-like structure and display rich surface chemistry, and numerous functional groups are present on their surface. Several functional groups, such as amide, aldehyde, ketone, carboxylic acid, alkene, hydroperoxide, nitroso, carbonate ester, and alcohol groups, are present on nanodiamond surfaces, assisting in their further functionalization for desired applications ( Fig. 20 ). 226
Furthermore, nanodiamond surfaces can be homogenized with a single type of functional group according to the application requirements. 227 The use of nanodiamond particles as a reinforcing material in polymer composites has attracted great attention for improving the performance of polymer composite materials. The superior mechanical properties and rich surface chemistry of nanodiamonds have made them a superior material for tuning and reinforcing polymer composites. Nanodiamonds might operate via changing the interphase properties and forming a robust covalent interface with the matrix. 228 Nanodiamond (ND)-reinforced polymer composites have shown superior thermal stabilities, mechanical properties, and thermal conductivities. Nanodiamonds have shown great potential for energy storage applications. 229 Nanodiamonds and their composites are also used in sensor fabrication, environmental remediation, and wastewater treatment. 230,231 Their stable fluorescence and long fluorescence lifetimes have made nanodiamonds useful for imaging and cancer treatment. For biomedical applications, the rational engineering of nanodiamond particle surfaces has played a crucial role in the carrying of bioactive substances, target ligands, and nucleic acids, resisting their aggregation. 232,233 Nanodiamonds have a great future in nanotechnology due to their amazing surface chemistry and unique characteristics.
Carbon quantum dots can be synthesized through several chemical routes. 241–245 Some methodologies for synthesizing carbon dots are described in Fig. 21 . 246–248 Carbon itself is a black material and displays low solubility in water. In contrast, carbon quantum dots are attractive due to their excellent solubility in water. They contain a plethora of oxygen-containing functional groups on their surface, such as carboxylic acids. These functional moieties allow for further functionalization with biological, inorganic, polymeric, and organic species.
Carbon quantum dots are also called carbon nano-lights due to their strong luminescence. 248 In particular, carbon quantum dots offer enhanced chemiluminescence, 249,250 fluorescent emission, 251 two-photon luminescence under near-infrared pulsed-laser excitation, 252 and tunable excitation-dependent fluorescence. 253 The luminescence characteristics of carbon quantum dots have been used to develop highly sensitive and selective sensors. In most cases, a simple principle is involved in sensing with luminescent carbon quantum dots: their photoluminescence intensity changes upon the addition of an analyte. 254 Based on this principle, several efficient sensors have been developed using carbon quantum dots. 255–257 They can be used as sensitive and selective tools for sensing explosives such as TNT. Recognition molecules on the surfaces of carbon quantum dots can help to sense targeted analytes. Amino-group-functionalized carbon quantum dot fluorescence is quenched in the presence of TNT through a photo-induced electron-transfer effect between TNT and primary amino groups. This quenching phenomenon can help to sense the target analyte ( Fig. 22 ). 258 Chiral carbon quantum dots (cCQDs) can exhibit an enantioselective response. The PL responses of cCQDs were evaluated toward 17 amino acids and it was found that the PL intensity of the cCQDs was only substantially enhanced in the presence of L -Lys ( Fig. 22 ). 254
Carbon quantum dots have received significant interest in the fields of biological imaging and nanomedicine ( Fig. 23 ). 239 Direct images of RNA and DNA are essential for understanding cell anatomy. Due to the limitations of current imaging probes, tracking the dynamics of these biological macromolecules is not an easy job. Recently, membrane-penetrating carbon quantum dots have been developed for the imaging of nucleic acids in live organisms. 259 It is important to note that most of the carbon quantum dots utilized to attain cell imaging under UV excitation emit blue radiation. Some biological tissue also emits blue light, specifically that involving carbohydrates, and this interferes with cell imaging carried out with blue-emitting CQDs. This seriously hinders their potential in the field of biomedical imaging. Due to this reason, researchers are focusing on tuning CQDs in a way that their emission peak is red-shifted to avoid interference. 260 Carbon quantum dots with yellow and green fluorescence have been reported for bioimaging purposes. 261,262 The suitable doping of carbon quantum dots can red-shift the emission to enhance the bioimaging effectiveness. 263 Doped carbon quantum dots are capable of biological imaging and display advanced capabilities for scavenging reactive oxygen species. 264
Carbon quantum dots demonstrate photo-induced electron transfer properties 265 that make them valuable for photocatalytic, light-energy conversion, and other related applications. 266 Carbon quantum dots enhance the activities of other photocatalysts to which they are attached. Carbon quantum dots, along with photocatalysts, provide better charge separation and suppress the regeneration of photogenerated electron–hole pairs. Moreover, the proper implantation of carbon quantum dots into photocatalysts can broaden the photo-absorption region. Implanted carbon quantum dots form micro-regional heterostructures that facilitate photo-electron transport. 267 The implantation of carbon quantum dots into g-C 3 N 4 can substantially enhance charge transfer and separation efficiencies, prevent photoexcited carrier recombination, narrow the bandgap, and red shift the absorption edge. 268 The intrinsic catalytic activity of polymeric carbon nitride is improved as a result of the nano-frame heterojunctions formed with the help of CQDs. 269
Carbon quantum dots offer many advantages over conventional semiconductor-based QDs and, thus, they have attracted considerable researcher attention. 244 Due to their remarkable features, they have shown importance in recent years in the fields of light-emitting diodes, nanomedicine, solar cells, sensors, catalysis, and bioimaging. 236
The production of carbon nanohorns has some obvious advantages over carbon nanotubes, such as the ability for toxic-metal-catalyst-free synthesis and large-scale production at room temperature. Carbon nanotube synthesis involves metal particles, and harsh conditions, such as the use of strong acids, are required to remove metallic catalysts. This process introduces many defects into CNT structures and may cause a loss of carbon material. 270 Carbon nanohorns possess a wide diameter compared to CNTs. CNHs possess good absorption capabilities and their interiors are also available after partial oxidation, which provides direct access to their internal parts. Heat treatment under acidic or oxidative conditions facilitates the facile introduction of holes into carbon nanohorns. Holes in graphene sheets of single-walled carbon nanohorns can be produced with O 2 gas at high temperatures. A large quantity of material can be stored inside CNH tubes. 274 The surface area of CNHs is substantially enhanced upon opening the horns to make their interiors accessible. 275 Carbon nanohorns have great potential for energy storage, 275 electrochemiluminescence, 276 adsorption, 277 catalyst support, 278 electrochemical sensing, 279 and drug delivery system 273 uses. CNHs as catalyst supports can provide a homogeneous dispersion of Pt nanoparticles ( Fig. 25 ). The current density of Pt supported on single-walled carbon nanohorns is double compared to a fuel cell made from Pt supported on carbon black. 280 Thus, carbon nanohorns provide a better uniform dispersion that facilitates a high surface area and better catalyst performance.
5.2. Nanoporous materials
In nanoporous materials, the size distributions, volumes, and shapes of the pores directly affect the performances of porous materials for particular applications. It has become a hot area of research to develop materials with precisely controlled pores and arrangements. Recent research has focused more on the precise control of the shapes, sizes, and volumes of pores to produce nanoporous materials with high performance. Several state-of-the-art reviews are present in the literature that focus explicitly on the synthesis, properties, advances, and applications of nanoporous materials. 85,287–289 Based on the materials used, nanoporous materials can be divided into three main groups: inorganic nanoporous materials; carbonaceous nanoporous materials; and organic polymeric nanoporous materials.
Inorganic nanoporous materials include porous silicas, clays, porous metal oxides, and zeolites. The generation of pores in the material can introduce striking features into the material that are absent in non-porous materials. Nanoporous materials offer rich surface compositions with versatile characteristics. Nanoporous materials exhibit high surface-to-volume ratios. Their outstanding features and nanoporous framework structures have made these materials valuable in the fields of environmental remediation, adsorption, catalysis, sensing, energy conversion, purification, and medicine. 284,290
Porous silica is a crucial member of the inorganic nanoporous family. Over the decades, it has generated significant research interest for use in fuel cells, chemical engineering, ceramics, and biomedicine. It is essential to note that specific morphologies and pore size diameters are required for each application, and these can be achieved via tuning during the synthesis process. Nanoporous silica offers two functional surfaces: one is the cylindrical pore surfaces, and the second is the exterior surfaces of the nanoporous silica particles. The surfaces of nanoporous silica can be easily functionalized for the desired applications. The nanoporous silica surface is heavily covered with many silanol groups that act as reactive sites for functionalization ( Fig. 26 ). 291,292 For biomedical applications, mesoporous silica has emerged as a new generation of inorganic platform materials compared to other integrated nanostructured materials. Several factors make it a unique material for biomedical applications: 293,294 (a) its ordered porous structure; (b) its tunable particle size; (c) its large pore volume and surface area; (d) its biocompatibility; (e) its biodegradation, biodistribution, and excretion properties; and (f) its two functional surfaces. For instance, ordered MCM-48 nanoporous silica was used for the delivery of the poorly soluble drug indomethacin. It has been found that surface modification can control drug release. 295 Mesoporous silica-based materials have emerged as excellent materials for use in sustained drug delivery systems (SDDSs), immediate drug delivery systems (IDDSs), targeted drug delivery systems (TDDSs), and stimuli-responsive controlled drug delivery systems (CDDSs). The drug release rate from mesoporous silica can also be controlled via introducing appropriate polymers or functional groups, such as CN, SH, NH 2 , and Cl. Researchers are currently focusing on developing MSN-based (MSN = mesoporous silica nanoparticle) multifunctional drug delivery systems that can release antitumor drugs on demand in a targeted fashion via minimizing the premature release of the drug ( Fig. 27 ). 296
Hierarchically nanoporous zeolites are a vital member of the nanoporous material family. They are crystalline aluminosilicate minerals whose structures comprise uniform, regular arrays of nanopores with molecular dimensions. The microporous structures of zeolites contain pores that are usually below 1 nm in diameter. In zeolites, the micropores are uniform in shape and size, and these pores can effectively discriminate between molecules based on shape and size. 297 Currently, based on crystallography, more than 200 zeolites have been classified. 298 Zeolites have been proved to be useful materials in the field of host–guest chemistry. In solid catalysis, about 40% of the entire solid catalyst field is taken up by zeolites in chemical industry. The excellent catalysis success of zeolites is based on their framework stability, shape-selective porosity, solid acidity, and ion-exchange capacity. Oxygen tetrahedrally coordinates with the Al atoms in most zeolite crystalline silicate frameworks, resulting in charge mismatch between the oxide framework and Al. Extra-framework Na + ions compensate for this charge mismatch. The Na + ions are exchangeable for other cations such as H + and K + . 298 The zeolite crystalline networks are remarkable in that they provide high mechanical and hydrothermal stabilities. The most crucial task facing the zeolite community is to find new structures with desired functions and apply them more effectively for different applications.
Apart from these inorganic porous materials, several other metal- and metal-oxide-based nanoporous materials have been introduced that are more prominent for use in electrode material, catalyst, photodegradation, energy storage, and energy conversion applications. 299–302 Nanoporous metal-based materials are famous due to the nanosized crystalline walls, interconnected porous networks, and numerous surface metal sites that provide them with unique physical/chemical properties compared with their bulk counterparts and other nanostructured materials. 303 For example, nanoporous WO 3 films were developed via tuning the anodization conditions for photoelectrochemical water oxidation. It has been observed that the morphology of the film strongly affected the photoelectrochemical performance. 304 Nanoporous alumina is also a unique material in the inorganic nanoporous family due to several aspects. Nanoporous alumina can be prepared in a controlled fashion with any size and shape in polyprotic aqueous media via the anodic oxidation of the aluminum surface. The parallel arrangement of pores on alumina can easily be controlled from 5 nm to 300 nm, and alumina is stable in the range of 1000 °C. The anodizing time plays a significant role in controlling the pore length. Nanoporous alumina membranes offer various unique properties, such as pores of variable widths/lengths, temperature stability, and optical transparency. Nanoporous alumina pores can be filled with magnetically and optically active elements to produce the desired applications at the nanoscale level. Photoluminescent alumina membranes can be produced via introducing cadmium sulfide, gallium nitride, and siloxenes inside nanoporous alumina using appropriate precursors. 305 Porous alumina also acts as an efficient support and template for the designing of other nanomaterials. Palladium nanowires, 306 high aspect ratio cobalt nanowires, 307 and highly aligned Cu nanowires 308 were developed using porous alumina as a template. Ni–Pd as a catalyst was supported on porous alumina for hydrogenation and oxidation reactions. 309 Nanoporous anodic alumina is also considered to be an efficient material for the development of biosensors due to the ease of fabrication, tunable properties, optical/electrochemical properties, and excellent stability in aqueous environments. 310
Nanoporous carbon-based materials are a hot topic in the field of materials chemistry. Nanoporous carbon materials have become ubiquitous choices in the environmental, energy, catalysis, and sensing fields due to their unique morphologies, large pore volumes, controlled porous structures, mechanical, thermal, and chemical stabilities, and high specific surface areas ( Fig. 28A ). 311 Nanoporous materials are found to be useful in the treatment of water. The separation of spilled oil and organic pollutants from water has emerged as a significant challenge. 312–314 The design of materials that can allow the efficient separation of organic, dye, and metal contaminants from water has become a leading environmental research area. 315,316 Nanoporous carbon can be derived from different natural and synthetic sources. 317–319 Nanoporous carbon foam can be derived from natural sources, such as flour, pectin, and agar, via table-salt-assisted pyrolysis. The agar-derived nanoporous carbon foam showed high absorption capacities, a maximum of 202 times its own weight, for oil and organic solvents. Air filtration paper developed from carbon nanoporous materials and non-woven fabrics has shown a filtration efficiency of greater than 99% ( Fig. 28B ). 320 Nanoporous carbon can also be produced from other porous frameworks, such as metal–organic frameworks. MOF- and COF-based materials are promising precursors for nanoporous carbon-based materials. The direct carbonization of amino-functionalized aluminum terephthalate metal–organic frameworks has produced nitrogen-doped nanoporous carbon that shows an adequate removal capacity of 98.5% for methyl orange under the optimum conditions. 321 Fe 3 O 4 /nanoporous carbon was also produced with Fe salts as a magnetic precursor and MOF-5 as a carbon precursor for removing the organic dye methylene blue (MB) from aqueous solutions. 322 The mesoporous carbon removal efficiency could be further enhanced via modifying or functionalizing the surface with various materials. Unmodified mesoporous carbon has shown a mercury removal efficiency of 54.5%. This efficiency can be substantially improved to 81.6% and 94% upon modification with the anionic surfactant sodium dodecyl sulfate and cationic surfactant cetyltrimethyl ammonium bromide (CTAB), respectively. 323
Ordered nanoporous carbon, CNTs, and fullerenes are extensively applied for energy and environmental applications. The complicated synthesis routes required for fullerenes and CNTs have slowed down the full exploitation of their potential for highly demanding applications. In comparison, the synthesis of highly ordered nanoporous carbon is facile, and the properties of ordered nanoporous carbon are also appealing for energy and environmental applications. 311 CO 2 is a greenhouse gas, and its sustainable conversion into value-added products has become the subject of extensive research. A nitrogen-doped nanoporous-carbon/carbon-nanotube composite membrane is a high-performance gas-diffusion electrode applied for the electrocatalytic conversion of CO 2 into formate. A faradaic efficiency of 81% was found for the production of formate. 324 Nanoporous carbon materials modified with the non-precious elements P, S, N, and B have emerged as efficient electrode materials for use in the oxygen evolution reaction (OER), hydrogen evolution reaction (HER), oxygen reduction reaction (ORR), batteries, and fuel cells. 311,325–327
Nanoporous polymers, including nanoporous coordination polymers and crystalline nanoporous polymers, have emerged as impressive nanoporous materials. 328 Nanoporous polymers have many applications, and these materials are extensively being evaluated for gas separation and gas storage. The great interest in these applications arises from the presence of pores providing an exceptionally high Brunauer–Emmett–Teller (BET) surface area. Recently, new classes of metal organic framework and covalent organic framework porous materials have been reported that have shown exceptionally high and unprecedented surface areas. For instance, in 2010, a MOF was reported with a surface area of 6143 m 2 g −1 ; 329 in 2012, a MOF was reported with a surface area greater than 7000 m 2 g −1 ; 330 and in 2018, a MOF (DUT-60) was reported with a record surface area of 7836 m 2 g −1 . 331 Mesoporous DUT-60 has also shown a high free volume of 90.3% with a density of 0.187 g cm −3 . 331
Due to their exceptionally high surface areas and porous networks, these MOFs and COFs are ideal for gas storage. Air separation and post-combustion CO 2 capture have become integral parts of mainstream industries related to the energy sector in order to avoid substantial economic penalties. Due to the inefficiencies of available technology and the critical importance of this area, earnest efforts are being made to design gas-selective porous materials for the selective adsorption of desired gases. Nanoporous MOF- and COF-based materials can significantly capture CO 2 and help reach zero or minimum CO 2 emission levels. For instance, nanoporous fluorinated metal–organic frameworks have shown the selective adsorption of CO 2 over H 2 and CH 4 . 332 Hasmukh A. Patel et al. developed N 2 -phobic nanoporous covalent organic polymers for the selective adsorption of CO 2 over N 2 . The azo groups in the framework rejected N 2 , leading to CO 2 selectivity. 333 Nanoporous polymers that are superhydrophobic in nature can also be used for volatile organic compounds and organic contaminants. 334 Nanoporous polymers, due to the presence of a porous network, have been considered as highly suitable materials for catalyst supports. Furthermore, organocatalytic functional groups can be introduced pre-synthetically and post-synthetically into solid catalysts. 335
Nanoporous polymeric materials are amazingly heading towards being extremely lightweight with exceptionally high surface areas. These high surface areas and the fine-tuning of the nanopores has made these nanoporous materials, specifically MOFs and zeolites, ideal support materials for encapsulating ultrasmall metal nanoparticles inside void spaces to produce nanocatalysts with exceptionally high efficiencies. 336 In the coming years, more exponential growth of nanoporous materials is expected in the energy, targeted drug delivery, catalysis, and water treatment fields.
5.3. Ultrathin two-dimensional nanomaterials beyond graphene
However, from a material synthesis standpoint, a graphite-like layered form of Si does not exist in nature and there is no conventional exfoliation process that can generate 2D silicene, although single-walled 351 and multi-walled 352 silicon nanotubes and even monolayers of silicon have been synthesized via exfoliation methods. 353 Forming honeycomb Si nanostructures on substrates like Ag(001) and Ag(110) via molecular beam deposition, so-called “epitaxial growth”, was then proposed as a method for the architectural design of silicene sheets. 354–356 The successful synthesis of a silicene monolayer was first achieved on Ag(111) and ZrB 2 (0001) substrates in 2012; 357,358 later, various demonstrations were made using Ir(111), ZrB 2 (001), ZrC(111), and MoS 2 surfaces as the silicene growth substrates. 359–361 Despite various extensive studies to date involving the “epitaxial growth” of silicene on different substrates and investigations of the electronic properties, 357,362–364 the limited nanometer size, difficulties relating to substrate removal, and air stability issues have substantially impeded the practical applications of silicene. Bearing in mind all these known difficulties, Akinwande and co-workers recently reported a growth–transfer–fabrication process for novel silicene-based field-effect transistor development that involved silicene-encapsulated delamination with native electrodes. 365 An etch-back approach was used to define source/drain contacts in Ag film. Without causing any damage to the silicene, a novel potassium-iodide-based iodine-containing solution was used to etch Ag, avoiding rapid oxidation, unlike other commonly used Ag etchants. The results demonstrated that this was the first proof-of-concept study confirming the Dirac-like ambipolar charge transport predictions made about silicene devices. Comparative studies with a graphene system, the low residual carrier density, and the high gate modulation suggested the opening of a small bandgap in the experimental devices, proving that silicene can be considered a viable 2D nanomaterial beyond graphene.
Nonetheless, the synthesis of silicene on a large-scale is greatly limited, as “epitaxial growth” is the only promising method for obtaining high-quality silicene, and this presents an enduring challenge in relation to silicene research and development. Xu and co-workers recently introduced liquid oxidation and the exfoliation of CaSi 2 as a means for the first scalable preparation of high-quality silicene nanosheets. 366 This new synthetic strategy successfully induced the exfoliation of stacked silicene layers via the mild oxidation of the (Si 2 n ) 2 n layers in CaSi 2 into neutral Si 2 n layers without damage to the pristine silicene structure ( Fig. 29 ). The selective oxidation of pristine CaSi 2 into free-standing silicene sheets without any damage to the original Si framework was carried out via exfoliation in the presence of I 2 in acetonitrile solvent. Furthermore, the obtained silicene sheets yielded ultrathin monolayers or layers with few-layer thickness and exhibited excellent crystallinity. This 2D silicene nanosheet material was extensively explored as a novel anode, which was unlike previously developed silicon-based anodes for lithium-ion batteries. It displayed a theoretical capacity of 721 mA h g −1 at 0.1 A g −1 and superior cycling stability of 1800 cycles. Overall, during the last decade, silicene has been widely accepted as an ideal 2D material with many fascinating properties, suggesting great promise for a future beyond graphene.
Like other 2D materials, MXenes exhibit crystal geometry with a hexagonal close-packed structure based on the equivalent MAX-phase precursor, and the close-packed structure is formed from M atoms with X atoms occupying octahedral sites. 371 According to the formula, there are three representative structures of MXenes: M 2 XT x , M 3 X2T x , and M 4 X3T x . In these combinations, X atoms are formed with n layers, whereas M atoms have n + 1 layers ( Fig. 30 ). 372 Apart from graphene, MXenes are considered the most dynamic developing material, and they have incredible innovation potential amongst typical 2D nanomaterials because of their remarkable properties, such as hydrophilicity, conductivity, considerable adsorption abilities, and catalytic activity. These vital properties of MXenes suggest their use for various potential applications, including in the photocatalysis, electrocatalysis, 373,374 energy, 375 membrane-based separation, 376,377 and biological therapy 378 fields. In this section, we focus on describing new developments relating to MXenes that are utilized for electrocatalytic and energy storage applications, competing as alternatives to graphene materials.
Interestingly, due to the presence of abundant terminal groups, mainly –O, –OH, and –F, and their modifying nature, MXenes can exhibit outstanding hydrophilic properties and high conductivity and charge carrier mobility, making them a very attractive material for various electrocatalytic applications, such as the hydrogen evolution reaction, oxygen evolution reaction, oxygen reduction reaction, nitrogen reduction reaction, and CO 2 reduction reaction. To further increase their electrocatalytic activities, recent works involving MXenes have included incorporation with CNTs, 379 g-C 3 N 4 , 380 FeNi-LDH, 381 NiFeCo-LDH, 382 and metal–organic frameworks. 383
Cho and co-workers designed and developed MXene–TiO 2 2D nanosheets via the surface oxidation of MXene with defect-free control. These MXene–TiO 2 2D nanosheets were successfully implemented in nano-floating-gate transistor memory (NFGTM) providing a floating gate ( i.e. , multilayer MXene) and tunneling dielectric ( i.e. , the TiO 2 layer). A process of oxidation in water further represented a cost-effective and environmentally benign method, as depicted in Fig. 31 . The MXene NFGTM with an optimal oxidation process displayed exceptional nonvolatile memory features, having a great memory window, high programming/erasing current ratio, long term retention, and high durability. 384
There have been some exciting reports on 2D materials from the pnictogen family, particularly phosphorene. Recently, more attention has also been given to the remaining group 15 elements, 390 with the novel 2D materials arsenene, antimonene, and bismuthene being obtained from the key elements arsenic, antimony, and bismuth, respectively. It is reported that 2D monolayers of group 15 elements, including phosphorene allotropes, have five distinct honeycomb (α, β, γ, δ, and ε) and four distinct non-honeycomb (ζ, η, θ, and ι) structures, as depicted in Fig. 32 . Dissimilar crystal orientations were found for single-layered As, Sb, and Bi. Zeng and co-workers also reported comprehensive density functional theory (DFT) computations that proved the energetic stability and broad-range application of these materials in 2D semiconductors. 391 Previously, following theoretical predictions, Wu and co-workers successfully demonstrated that α-phosphorene showed lowest energy configurations in both honeycomb and non-honeycomb nanosheets. 392 In contrast, Zeng and co-workers proved that the buckled forms of 2D sheets of As, Sb, and Bi allotropes are the most stable structures, particularly their β phases. 391
Among monolayer group 15 family materials, 2D sheets of arsenic (As) and antimony (Sb) have gained considerable attention from researchers. 393,394 Studies have shown that As and Sb exhibit better stability than black phosphorus; they are highly stable at room temperature and less reactive to air, likely inhibiting the oxidization process. 395–398 Nevertheless, it has been demonstrated that the oxidation process is perhaps favorable for fine-tuning the electronic properties; increases in the indirect band gaps ranging from 0 to a maximum of 2.49 eV are found in free-standing arsenene and antimonene semiconductors. 399–403 Simultaneously, arsenene and antimonene can also be transformed into semiconductors with direct band gaps. These two 2D nanosheets can be used to design mechanical sensors, moving beyond common electronic and optoelectronic applications. These two extraordinary 2D nanosheets have been studied for their structural–property relationships via first-principles methods. 403–405
Continuing the characterization and structural property studies of arsenene carried out by Kamal 404 et al. and Zhang 403 et al. , Anurag Srivastava and co-workers analyzed applications of arsenene to explore the possibility of improving sensor devices that can be utilized to detect ammonia (NH 3 ) and nitrogen dioxide (NO 2 ) molecules. 406,407 They investigated the affinities of NH 3 and NO 2 molecules for pristine arsenene sheets, examining the binding energies, bonding distances, density distributions, and current–voltage features. The results showed that arsenene 2D sheets are highly durable, with significant electronic charge transfer. They also considered germanium-doped arsenene and characterized the 2D lattice based on molecular affinity relationships with respect to the dopant.
However, the incorporation of any dopants into 2D nanomaterials not only results in experimental difficulty but it also lowers the stability of 2D materials. 408 Recently, Dameng Liu and co-workers reported the electronic structures, focusing on band structures, band offsets, and intrinsic defect properties, of few-layer arsenic and antimony. 409 The spontaneous oxide passivation layer that is formed naturally on pristine antimonene provides excellent stability. 410 Very recently, Stefan Wolff and co-workers conducted DFT calculations on various single or few-layer antimony oxide structures to describe the stoichiometry and bonding type. Interestingly, the samples exhibited various structural stabilities and electronic properties with a wide range of direct and indirect band gaps. Showing band gaps between 2.0 and 4.9 eV, these 2D layers of antimonene exhibited the potential to be used as insulators or semiconductors. 411 The same group also analyzed Raman spectra and discussed identifying the predicted antimonene oxide structures experimentally. The enduring task of exploring the utility of antimonene has boosted recent research interest in 2D nanomaterials due to the broad range of potential applications, such as their use in electrochemical sensors, 412,413 stable organic solar cells, 414 and supercapacitors 415 to name a few.
The 2D MOF nanosheets are also evaluated for the development of high-performance power-storage devices. For example, Li et al. 427 recently reported two novel Mn-2D MOFs and Ni-2D MOFs as anode materials for rechargeable lithium batteries. The Mn-based ultrathin metal–organic-framework nanosheets, due to thinner nanosheets, a higher specific surface area, and smaller metal ion radius, had structural advantages over Ni-based ultrathin metal–organic-framework nanosheets. Due to these features, the Mn-based ultrathin metal–organic-framework nanosheets displayed a high reversible capacity of 1187 mA h g −1 at 100 mA g −1 for 100 cycles and a rate capability of 701 mA h g −1 even at 2 A g −1 .
The expensive metal oxides utilized in the catalytic process can be replaced in due course by 2D-MOF-based nanosheets with exposed metal sites that impart an adjustable pore structure, ultrathin thickness, a high surface-to-volume atom ratio, and high design flexibility. As a result, 2D-MOFs have extensively been explored for various electrocatalytic applications, including the hydrogen evolution reaction (HER), oxygen evolution reaction (OER), oxygen reduction reaction (ORR), and carbon dioxide reduction reaction (CO 2 RR). For example, Marinescu et al. 428 combined cobalt dithiolene species with benzenehexathiol (BHT) and yielded 2D-MOFs capable of acting as electrocatalysts for the HER in water ( Fig. 34 ). In the presence of 2D-MOF sheets, a high current density of 41 mA cm −2 , at −0.8 V vs. SHE and a pH value of 1.3, is observed. Similarly, Feng et al. 429 also developed single-layer Ni-based 2D-MOF sheets that are highly effective for electrocatalytic hydrogen evolution. Later, Patra et al. 430 reported similar 2D sheets from covalent organic frameworks (2D-COFs) as metal-free catalysts for HER applications. 2D-MOFs are also being explored as active catalysts for the OER process. For example, Xu et al. 431 reported the preparation of 2D Co-MOF sheets using polyvinylpyrrolidone as a surfactant under mild solvothermal conditions. These novel 2D Co-MOFs displayed ultrathin nanosheets with many surface-based metal active sites, improving the overall OER performance.
Interestingly, experimental electrochemical measurement data showed that Co-MOF sheets offer a low overpotential ( i.e. , 263 mV at 10 mA cm −2 ). Similarly, Wang et al. 432 also reported that double-metal 2D-sheets (2D NiFe MOFs) consisting of a very ultrathin structure with a thickness of ∼10 nm further offer a low overpotential of 260 mV at 10 mA cm −2 . In other reports, Zhang et al. 433 successfully performed the OER process with ultrathin 2D-MOF sheets prepared via electrochemical and chemical exfoliation strategies.
Recent work on the catalytic activity of 2D-MOFs has also been reported in relation to the ORR and CO 2 RR because of their layered crystal structures and high-volume modifiable porous structures. For example, Dincă et al. 434 demonstrated that ultrathin layered conductive sheets of the 2D-MOF Ni 3 (HITP) 2 (HITP = 2,3,6,7,10,11-hexaiminotriphenylene) could actively be utilized as a catalyst in an alkaline medium for the ORR process. These 2D-MOF sheets show high stability while retaining 88% of the initial current density over 8 h at 0.77 V vs. RHE. In another report, through fabricating Co x Zn 2− x (bim) 4 2D-sheets as precursors, Zhao et al. 435 successfully synthesized cobalt nanodots (Co-NDs) with bimetallic Co x Zn 2− x (bim) 4 nanosheets encapsulating few-layer graphene (Co@FLG). For the CO 2 RR, a cobalt–porphyrin-containing 2D-MOF was achieved for the selective electrochemical reduction of CO 2 to CO with enhanced stability by Peidong Yang and co-workers. 436 The results further proved that these thin-film catalysts have the highest selectivity for CO ( i.e. , 76%) at −0.7 V vs. RHE with the little-to-no substantial decrease in activity over 7 h at −0.7 V vs. RHE, and 16 mL of CO was produced. Besides, like many other porous materials, 2D-MOFs were also shown to be a supporting platform for catalytic nanoparticles because of their high specific surface areas and favorable porosity distributions. To this end, an example can be noted from Wang et al. 437 reporting that fine porous MOF-5 nanosheets can be utilized to immobilize Pd nanoparticles.
5.4. Metal-based nanostructured materials
As discussed, catalysis is one of the main uses of metal-based nanostructured materials. A continuous increase in the demand for energy, the rapid depletion of conventional energy reservoirs, and rising concerns over the emission of CO 2 have increased the challenges and urgency in the energy field. 460 Metal-based nanostructured materials are extensively being explored to produce alternative clean and renewable energy sources. A range of metal-based nanomaterials has been evaluated and is under consideration for developing robust electrodes that can be effectively applied to water splitting, batteries, and solar cells.
High energy demands have led to more pressure to improve the performances of existing highly demanded lithium-ion batteries. Researchers have focused on improving their lifetimes, sizes, and safety. 462 Nanostructured metal-oxide-based materials are promising electrode materials for use in high-performance charge-storage devices. A metal-based nanostructured electrode is evaluated as both the anode and cathode to overcome the challenges of conventional electrodes. 463 In a conventional LIB, LiCoO 2 was used as the cathode material. Controlled morphology plays a crucial role in determining the performance of a material. Powder composed of spherical particles of LiNi 0.8 Co 0.2 O 2 showed a higher tap density compared to irregular particles and the material substantially improved the power density of secondary lithium batteries. 464 Hierarchical nanostructures of metal-based oxides (such as 3D hierarchical ZnCo 2 O 4 nanostructures) have emerged as a new trend for the development of high-capacity electrodes for lithium-ion batteries. 465 Since their commercialization by Sony in the early 1990s, LIBs have achieved tremendous success in bringing portable electronic devices to the market. However, their sustainable development on the grid-scale is hampered due to limited Li resources in nature, and this is causing a continuous increase in cost. 466 Sodium-ion batteries are in the spotlight to replace powerful lithium-ion batteries due to the widespread availability of sodium and its lower cost compared with lithium. 467 It is essential to note that, in terms of energy densities for SIBs, it is difficult to bypass LIBs because of the low standard electrochemical potential and higher weight of Na. SIBs could be proved to be ideal for those applications where cost is a critical factor compared to energy density. 466
SIBs also operate similarly to LIBs, based on an intercalation mechanism. SIBs also consist of cathode and anode electrodes separated through an electrolyte. During the charging process, sodium ions are extracted from the cathode and inserted into the anode via the electrolyte. In the discharging process, the electrons leave the anode through an external circuit to reach the cathode, providing electricity to the load, whereas Na + moves to the cathode during this process. The radius of Na + (1.02 Å) is greater than that of Li + (0.76 Å), making it challenging to intercalate into electrode materials. 468 Thus, appropriate electrode materials are required in which fast Na-ion insertion and extraction is possible. However, SIBs are suffering from a lack of appropriate electrode materials. It is important to develop electrode materials that have enough interstitial space within their crystallographic structures and better electrochemical performance. Among the various proposed electrode materials, Na x MO 2 layered transition-metal oxides (M = V, Fe, Cu, Co, Ni, Cr, Mn, and their combinations) are considered to be promising electrode materials for SIBs. Layered metal oxides are considered to be promising electrode materials due to their facile scalable synthesis, simple structures, appropriate operating potentials, and high capacities. 469,470 Large volume expansion and poor kinetics during the charge–discharge process can severely affect the cyclability and performance of SIBs. One of the effective strategies to deal with the mechanical stress triggered by large volume changes is the design of hollow or porous structures. In response, three-dimensional network-based Sb 2 O 3 @Sb composite anode materials can help to relieve the volume-change-related stress through their uniform porous networks and provide better transportation channels for Na + . 471
The large volume expansion of electrodes can also be buffered via designing 2D metal-oxide materials with large interlayer spacing. The ultrathin nanosheets provide high reversible capacity with enhanced cycling stability and contribute to providing reaction sites for electrons/ions, decreasing the diffusion distance, providing effective diffusion channels, and facilitating fast charge/discharge for sodium and lithium. 2D SnO nanosheet anodes were evaluated for SIBs. The capacity and cyclic stability improved, as the number of atomic SnO layers is decreased in the sheets. 472 Sb is a promising anode material, but during the sodiation/desodiation processes, huge volume expansion of 390% is observed, which hinders its practical use. Nanostructured Sb in the form of nanorod arrays with large interval spacing displays the great capacity to accommodate volume changes during cycling. 473 A comparison of various nanostructured metal-based electrodes for various charge storage purposes is shown in Table 3 . Overall, well-structured metal or metal-based oxide nanomaterials have the capacity to resolve current issues relating to charge storage devices.
Recently, an immense focus of research has been to produce H 2 fuel via water-splitting to replace conventional fossil fuels. This will help to eliminate emissions from the use of carbonaceous species. 484 Electrochemical method are considered simple water splitting approaches, as these methods only require an applied voltage and water as inputs to produce hydrogen fuel. 485 The coupling of solar irradiation to electrochemical water splitting has enhanced the performance and reduced the process cost. Due to these reasons, this has become a hot area of research. 486 During water electrolysis, H 2 is produced through the hydrogen evolution reaction at the cathode and O 2 is produced through the oxygen evolution reaction at the anode. However, water splitting is not so straightforward, and it requires an efficient catalyst that can facilitate the splitting of water. Metal- and metal-oxide-based catalysts are extensively being explored for water splitting. For the HER reaction, Pt-based catalysts are found to be suitable, whereas for OER reactions, Ir-/Ru-based compounds are found to be benchmark catalysts. Scarcity and high cost have limited the widespread use of these metals. The barrier of noble-metal cost can be mitigated through developing noble-metal nanostructured surfaces that produce more active sites or via depositing monolayers of noble metals on low-cost materials. The alloying of noble metals with other metals has enhanced site-specific activity. 484 At present, more focus is being placed on developing noble-metal-free catalysts for water splitting. 485 Usually, an efficient electrocatalyst is characterized by: 487 a low overpotential; high stability; low production costs; and high electrocatalytic activity.
The nano-structuring of catalysts is an effective tool to boost their surface areas. The electrolysis of water occurs at the surface of a catalyst, and nanostructured catalysts provide more active sites and the better diffusion of ions and electrolytes. 484 Non-noble metals that are under observation for the development of HER electrocatalysts include nickel (Ni), tungsten (W), iron (Fe), molybdenum (Mo), cobalt (Co), and copper (Cu). 487 For instance, a noble metal-free catalyst, carbon-decorated Co 3 O 4 nanoarrays on carbon paper, required a small overpotential of 370 mV to reach a current density of 10 mA cm −2 . It can maintain a current density of 100 mA cm −2 for 413.8 h and 86.8 h under alkaline and acidic conditions, respectively. 488
Metal-based semiconductor materials play a crucial role in a range of applications. For photoelectrochemical water splitting, the semiconductor material plays a central role in the solar-to-hydrogen conversion efficiency. Some critical features are prerequisites when it comes to selecting the right semiconductor material for the photoelectrochemical splitting of water: 489 an extraordinary capacity to absorb visible light; an appropriate bandgap; suitable valence and conduction band positions; commercial feasibility; and chemical stability.
For an ideal semiconductor for water splitting, the valence band and conduction band edge positions must straddle the oxidation and the reduction potentials of water. Metal oxides have received significant attention among semiconductors due to their wide band gap distributions, remarkable photo-electrochemical stabilities, and favorable band edge positions. 490 Semiconductor-based photoelectrodes become excited upon light irradiation, and electrons from the valence band move to the unoccupied conduction band. Some of the generated electrons at the cathode surface reduce protons to hydrogen gas, whereas holes at the photoanode produce oxygen gas via water splitting. 490 As a result, various nanostructured metal oxides can be used as photoelectrode materials, such as WO 3 , 491 Cu 2 O, 492 TiO 2 , 493 ZnO, 494 SnO 2 , 495 BiVO 4 , 496 and α-Fe 2 O 3 , 490 for the efficient splitting of water. As discussed, the nano-structuring of semiconductors can significantly impact the electrode photoelectrochemical performance during water splitting.
Metal-based nanomaterials have been used for the development of sensitive sensors. These metal-based sensors can replace the complex and expensive instruments that are conventionally used for the sensing of analytes. Metal-oxide-based sensors have the interesting characteristics of low detection limits, low cost, high sensitivity, and facile operation. 497 Mostly, semiconducting metal-oxide-based sensors are used for the sensing of toxic, flammable, and exhaust gases. Semiconductor metal oxides with a size in the range of 1–100 nm have been significantly investigated as gas sensors due to their size-dependent properties. The geometry and size of a nanomaterial can considerably affect the hole and electron movement in semiconductors. 498 The surface-to-volume ratio and surface area are substantially enhanced at the nanoscale level, and this is amazingly beneficial for sensing. Chemiresistive semiconducting metal oxides are potential candidates for gas sensing due to the following features: 499 rapid response times; fast recovery times; low cost; simple electronic interfaces; user-friendliness and low maintenance; and abilities to sense a wide range of gases.
Electrode materials decorated with metal- or metal-oxide-based nanostructured materials have shown better responses and selectivity for determining various analytes over conventional electrode materials. The nano-sized metal structures act as an electrocatalyst and electronic wires to provide rapid electron transfer between the transducers and analyte molecules. 500 The electrochemical redox reaction of H 2 O 2 can be improved via the thermally controlled anchoring of Pt NPs on the electrode surface. 501
Currently, researchers are not just concentrating on the development of randomly shaped nanomaterials; instead, they are very focused on and interested in the rational design of materials with controlled nano-architectures for boosting their performances for specific applications. As a result, extensive research has been carried out to develop metal-based materials with controlled dimensions to achieve better catalytic responses. Particle morphology is a crucial factor in the performance of nanomaterials for specific applications. Laifa Shen et al. rationally designed an electrode architecture via growing mesoporous NiCo 2 O 4 nanowire arrays on carbon textiles, which boosted the electrode performance ( Fig. 37 ). 474
The same materials with different morphologies can produce different outcomes. For instance, MnO 2 nanoflowers have provided high initial sodium-ion storage capacity compared with MnO 2 nanorods. 481 Radha Narayanan and Mostafa A. El-Sayed have analyzed various nanoscale morphologies of Pt, such as tetrahedral, cubic, and near-spherical nanoparticles. The highest rate constant is observed with tetrahedral nanoparticles and the lowest rate constant was observed with cubic nanoparticles, whereas spherical nanoparticles exhibited an intermediate rate constant during catalysis. 502 Xiaowei Xie et al. found that Co 3 O 4 nanorods show high activity compared to conventional Co 3 O 4 nanoparticles for the low-temperature oxidation of CO. 503 The catalytic activity of metal-based nanomaterials is strongly affected by their shape. 504 Shape-defined mesoporous materials (TiO 2 ) have shown superior photoanode activities ( Fig. 38 ). 505 As a result, in the literature, several nanostructured morphologies of metal-based materials, such as nanotubes, 506,507 nanorods, 508,509 nanoflowers, 510 nanosheets, 511 nanowires, 512 nanocubes, 513 nanospheres, 514,515 nanocages, 516 and nanoboxes, 517 have been reported for a range of applications.
Hollow nanostructures have surfaced as an amazing class of nanostructured material, and they have received significant attention from researchers. Hollow nanostructures have the unique features of: 518,519 low density; abundant inner void spaces; large surface areas; and the ability to act as nanoscale containers with high loading capacity, nanoreactors, and nanocarriers.
Various metal-based hollow nanostructures, such as hollow SnO 2 , 520 hollow palladium nanocrystals, 521 Co–Mn mixed oxide double-shell hollow spheres, 521 hollow Cu 2 O nanocages, 522 three-dimensional hollow SnO 2 @TiO 2 spheres, 523 hollow ZnO/Co 3 O 4 nano-heterostructure, 524 triple-shell hollow α-Fe 2 O 3 , 525 and hierarchical hollow Mn-doped Ni(OH) 2 nanostructures, 526 have been developed for various applications. The presence of nanoscale hollow interiors and functional shells imparts them with great potential for gas sensing, catalysis, biomedicine, energy storage, and conversion. 519
From this discussion, it can be concluded that metal-based nanostructured materials have great potential compared to their bulk counterparts. The conversion of materials to the nanoscale is not enough to achieve high performance with better selectivity. Now, research is switching from conventional nanomaterials to more advanced and smartly designed nanomaterials. In modern research, nanomaterials are being designed with better-controlled morphologies and regulated features.
5.5. Core–shell nanoparticles
A spherical nanoparticle core–shell nanostructure is a practical way to introduce multiple functionalities on the nanoscopic length scale. 528 The properties arising from the core or shell can be different, and these properties can be tuned via controlling the ratio of the constituent materials. The shape, size, and composition play a critical role in tuning the core–shell nanoparticle properties. 529 The shell material can help to improve the chemical and thermal stabilities of the core material. The core–shell design has become effective where an inexpensive material cannot be used directly due to its instability or easily oxidizable nature. The core can consist of an easily oxidizable inexpensive metal, whereas the shell might consist of noble metals, oxides, polymers, or silica. 530 For instance, magnetic nanoparticles when prepared can be sensitive toward air, acids, and bases. Magnetic nanoparticles can be protected via coating with organic or inorganic shells. 528
Core–shell metal nanoparticles are an emerging nanostructured material with great potential in the fields of energy and catalysis. 531 The first report of core–shell nanoparticles (2007) for supercapacitor applications consisted of a polyaniline/multi-walled-carbon-nanotube composite (PANI/MWNTs). 532 Metal-based core–shell structured nanoparticles have shown enhanced catalytic performance due to their shape-controlled properties. 533 Ming-Yu Kuo et al. developed Au@Cu 2 O core–shell particles with controllable shell thicknesses that acted as a dual-functional catalyst. The shell thickness of Cu 2 O increased with an increasing concentration of Cu 2+ precursor. The thicknesses of the shells of Au@Cu 2 O-1.5 (12.2 ± 1.7 nm), Au@Cu 2 O-2 (13.2 ± 1.8 nm), Au@Cu 2 O-3 (18.2 ± 2.2 nm), and Au@Cu 2 O-4 (20.8 ± 2.5 nm) due to various concentrations are shown in Fig. 40 . 534 A NiO@SiO 2 core–shell catalyst provided a higher yield of acrylic acid from acetylene hydroxycarbonylation. 535 Core–shell architecture can be used to prevent active metal nanoparticles from oxidation during operation. For instance, a plasmonic photocatalyst was developed that consisted of silver nanoparticles embedded in titanium dioxide. The direct contact of Ag with TiO 2 could lead to its oxidization; this is prevented via developing core–shell architecture in which Ag is used as the core and SiO 2 is used as a shell to protect it. 536 Another excellent option is to replace an expensive core with a non-noble metal to reduce the core–shell cost while using a thin layer of a noble metal that consumes a small amount of metal as the shell. This will ensure the prolonged stability of the catalyst during operation. 533 Overall, core–shell morphologies provide better catalytic activity due to the synergistic effect of the metallic core–shell components. 152
Among the several classes of nanomaterials, core–shell nanoparticles are found to be more promising for different biomedical applications. For instance, magnetic nanoparticles are considered to be useful for biomedical applications due to the following reasons: (a) aggregation is prevented due to superparamagnetism; (b) delivery and separation can be controlled using an external magnetic field; (c) they can be appropriately dispersed; and (d) there is the possibility of functionalization. A range of magnetic nanoparticles is available, such as NiO, Ni, Co, and Mn 3 O 4 . The most famous example is iron oxide, but uncoated iron oxides are unstable under physiological conditions. This may result in controlled drug delivery failure due to improper ligand surface binding and the promotion of the formation of harmful free radicals. Therefore, the formation of shells around magnetic nanoparticles has tremendous significance for biomedical applications. 537 One of the approaches is to use gold shells on magnetic nanoparticles. Au NPs are also called surface plasmons and they substantially enhanced the absorption of light in the visible and near-infrared regions. Thus, coating magnetic nanoparticles with a Au shell can result in a core–shell nanostructure that displays both optical and magnetic functionality in combination. 529
Numerous biocompatible core–shell nanoparticles are being developed for photothermal therapy, as core–shell materials are found to be useful for photothermal therapy. Hui Wang et al. have developed bifunctional core–shell nanoparticles for dual-modal imaging-guided photothermal therapy. The core–shell nanoparticles consist of a magnetic ∼9.1 nm core of Fe 3 O 4 covered by an approximately 3.4 nm fluorescent carbon shell. The Fe 3 O 4 core leads to superparamagnetic behavior, whereas the carbon shell provides near-infrared (NIR) fluorescence properties. The bifunctional nanoparticles have shown dual-modal imaging capacity both in vivo and in vitro . The iron oxide–carbon core–shell nanoparticles absorbed and converted near-infrared light to heat, facilitating photothermal therapy. 538 Au-Based core–shell structures are also being prepared for photothermal therapy. Bulk gold is biocompatible, but Au NPs can accumulate in the spleen and liver, causing severe toxicity. Koo Chul Kwon et al. have developed Au-NP-based core–shell structures that did not result in any gross or histological lesions in the major organs of mice, which revealed that this is a potent and safe agent for photothermal cancer therapy. The core–shell nanoparticles consisted of proteinticle/gold (PGCS-NP) and were developed via proteinticle surface engineering. PGCS-NP was injected intravenously into mice with tumors, and the injected core–shell nanoparticles successfully reached the EGFR-expressing tumor cells. The tumor size was significantly reduced upon exposure to near-infrared laser irradiation ( Fig. 41 ). No accumulation of Au NPs was observed in the mice organs, which indicated that PGCS-NP disassembled into many tiny gold dots, which were easily excreted by the kidneys and liver without causing any toxicity. 539 In another example, multifunctional Au@graphene oxide nanocolloid core@shell nanoparticles were developed, in which the core and shell consisted of gold and a graphene oxide nanocolloid, respectively. The developed core–shell structure showed multifunctional properties, allowing Raman bioimaging and photothermal/photodynamic therapy with low toxicity. 540 Apart from this, numerous other core–shell nanoparticles, such as polydopamine–mesoporous silica core–shell nanoparticles, 541 AuPd@PVP core–shell nanoparticles, 542 Au@Cu 2− x S core–shell nanoparticles, 543 bismuth sulfide@mesoporous silica core–shell nanoparticles, 544 and Ag@S-nitrosothiol core–shell nanoparticles, have been used for photothermal therapy. 545
Due to their unique features and the combination of properties from the shell and core, these core–shell nanoparticles have received considerable interest in many fields, ranging from materials chemistry to the biomedical field. For electrochemical reactions, the core–shell structure conductivity can be enhanced via conducting polymers, carbon materials, and metals. Core–shell nanoparticles as electrode materials showed better performance compared to single components. Most of the core materials are prepared via hydrothermal methods, and shells can be prepared via hydrothermal or electrodeposition methods. 546 Even though significant progress has been made relating to the synthesis methods of core–shell materials, a major challenge is the high-quality production of core–shell materials in more effective ways for required applications, specifically biomedical applications.
6. Challenges and future perspectives
(a) The presence of defects in nanomaterials can affect their performance and their inherent characteristics can be compromised. For instance, carbon nanotubes are one of the strongest materials that are known. However, impurities, discontinuous tube lengths, defects, and random orientations can substantially impair the tensile strength of carbon nanotubes. 547
(b) The synthesis of nanomaterials through cost-effective routes is another major challenge. High-quality nanomaterials are generally produced using sophisticated instrumentation and harsh conditions, limiting their large-scale production. This issue is more critical for the synthesis of 2D nanomaterials. Most of the methods that have been adopted for large-scale production are low cost, and these methods generally produce materials with defects that are of poor quality. The controlled synthesis of nanomaterials is still a challenging job. For example, a crucial challenge associated with carbon nanotube synthesis is to achieve chiral selectivity, conductivity, and precisely controlled diameters. 548,549 Obtaining structurally pure nanomaterials is the only way to achieve the theoretically calculated characteristics described in the literature. More focused efforts are required to develop new synthesis methods that overcome the challenges associated with conventional methods.
(c) The agglomeration of particles at the nanoscale level is an inherent issue that substantially damages performance in relevant fields. Most nanomaterials start to agglomerate when they encounter each other. The process of agglomeration may be due to physical entanglement, electrostatic interactions, or high surface energy. 550 CNTs undergo van der Waals interactions and form bundles, making it difficult to align or properly disperse them in polymer matrices. 159 Similarly, graphene agglomeration is triggered by the basal planes of graphene sheets due to π–π interactions and van der Waals forces. Due to severe agglomeration, the high surface areas and other unique graphene features are compromised. These challenges hinder the practical application of high-throughput electrode materials or composite materials for various applications. 551
(d) The efficiency of nanomaterials can be tuned via developing 3D architectures. 3D architectures have been tried with several nanomaterials, such as graphene, to improve their inherent features. 3D architectures of 2D graphene have provided high specific surface areas and fast mass and electron transport kinetics. This has become possible due to the combination of the exceptional intrinsic properties of graphene and 3D porous structures. 194,552 The combination of graphene and CNT assemblies into 3-D architectures has emerged as the most investigated nanotechnology research area. Porous architectures of other nanomaterials can be developed to enhance their catalysis performance through providing nanomaterial interior availability.
(e) 2D ultrathin materials are an outstanding class of nanomaterial with promising theoretical properties; however, very little experimental evaluation of these materials has been done, apart from the case of graphene. The synthesis and stability of 2D ultrathin materials are some of the major challenges associated with them. In the future, more focus is anticipated to be placed on their synthesis and practical utilization.
(f) Nanomaterial utilization in industry is being increased, and there is also demand for nanoscale material production at higher rates. Moreover, nanotechnology research has vast horizons; the exploration of new nanomaterials with fascinating features will continue and, in the future, more areas will be discovered. One of the significant concerns relating to nanomaterials that cannot be overlooked is their toxicity, which is still poorly understood, and this is a serious concern relating to their environmental, domestic, and industrial use. The extent to which nanoparticle-based materials can contribute to cellular toxicity is unclear. 553 There is a need for the scientific community to put efforts into reducing the knowledge gap between the rapid development of nanomaterials and their possible in vivo toxicity. A proper and systematic understanding of the interaction of nanomaterials with cells, tissues, and proteins is critical for the safe design and commercialization of nanotechnology. 14
The future of advanced technology is linked with advancements in the field of nanotechnology. The dream of clean energy production is becoming possible with the advancement of nanomaterial-based engineering strategies. These materials have shown promising results, leading to new generations of hydrogen fuel cells and solar cells, acting as efficient catalysts for water splitting, and showing excellent capacity for hydrogen storage. Nanomaterials have a great future in the field of nanomedicine. Nanocarriers can be used for the delivery of therapeutic molecules.
7. Conclusions
Conflicts of interest, acknowledgements.
- N. Taniguchi, Proc. Int. Conf. Prod. Eng. Issue PART II , 1974, 18–23.
- Nat. Nanotechnol. , 2019, 14 , 193 Search PubMed .
- M. S. Khan, V. Ranjan and A. Srivastava, Proc. – 2015 IEEE Int. Symp. Nanoelectron. Inf. Syst. iNIS 2015 , 2016, 248–251.
- Master's programmes in English
- For exchange students
- PhD opportunities
- All programmes of study
- Language requirements
- Application process
- Academic calendar
- NTNU research
- Research excellence
- Strategic research areas
- Innovation resources
- Student in Trondheim
- Student in Gjøvik
- Student in Ålesund
- For researchers
- Life and housing
- Faculties and departments
- International researcher support
Språkvelger
Course - master's thesis in nanotechnology - mol4901, course-details-portlet, mol4901 - master's thesis in nanotechnology, examination arrangement.
Examination arrangement: Master thesis Grade: Letter grades
Course content
The master's thesis is a project work that gives 30 credits. The project will usually be part of an ongoing research project related to nanomedicine / nanotechnology at the Faculty of Medicine and Health Sciences. Emphasize will be on learning to work systematically within the field of research, and that the student learns to aquire detailed knowledge within the field with the use of literature and practical work.
Learning outcome
After completing the course, the student should
- know how to formulate a precise research problem
- be able to scientifically test and answer a research problem
- have skills in preparing and analyzing study data
- know how to present a research problem and discuss the results critically by use of relevant scientific literature
- have skills in preparing the results in a scientific format with sound language an precise statements
- describe a scientific work in a clearly written report (master thesis)
Learning methods and activities
All students doing their master's thesis should conclude an agreement regarding counselling. This is done via the electronic form in Sharepoint. The standard contact period with the supervisor/-s is 25 hours. Any contact between supervisor/-s and student beyond this, depends on the supervisor/-s choice of supervision methodology. It is expected that the student works independently beyond the standard contact period. A national regulation for assessment of thesis in MST subjects has been introduced. Every thesis submitted as of 01/04/2014 will be graded according to this regulation. The regulations are described on the following website: https://innsida.ntnu.no/wiki/-/wiki/English/Description+of+grades+for+master+thesis Additional information can be found on this website (Norwegian only): https://innsida.ntnu.no/wiki/-/wiki/Norsk/Fordypningsordning+og+masteroppgave+ved+IBT+-+sivilingeni%C3%B8r.
Further on evaluation
Uses The Department of Physics', at NTNU, procedures for submission.
Specific conditions
Admission to a programme of study is required: Nanotechnology (MTNANO)
Required previous knowledge
The course is part of the masters program in nano technology (MTNANO). Requires approved / completed 9 semesters on the study program Nanotechnology, including a specialization project of 15 sp in the 9th semester.
Version: 1 Credits: 30.0 SP Study level: Second degree level
Term no.: 1 Teaching semester: SPRING 2024
Language of instruction: English
Location: Trondheim
- Medical Technology
- Nanotechnology
- Øyvind Halaas
- Anders Sundan
- Anne Mari Aukan Rokstad
- Geir Slupphaug
- Terje Espevik
- Therese Standal
- Vidar Beisvåg
Department with academic responsibility Department of Clinical and Molecular Medicine
Examination
Examination arrangement: master thesis.
- * The location (room) for a written examination is published 3 days before examination date. If more than one room is listed, you will find your room at Studentweb.
For more information regarding registration for examination and examination procedures, see "Innsida - Exams"
More on examinations at NTNU
- My Shodhganga
- Receive email updates
- Edit Profile
Shodhganga : a reservoir of Indian theses @ INFLIBNET
- Shodhganga@INFLIBNET
- Narsee Monjee Institute of Management Studies
- Department of Biological Sciences
Items in Shodhganga are licensed under Creative Commons Licence Attribution-NonCommercial-ShareAlike 4.0 International (CC BY-NC-SA 4.0).

- IEEE Xplore Digital Library
- IEEE Standards Association
- IEEE Spectrum Online
- More IEEE Sites
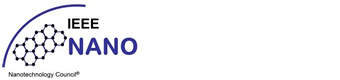
- The Nanotechnology Council
- NTC Travel Policy
- Frequently Asked Questions
- NTC Fellows
- 2023 Awardees
- 2022 Awardees
Call for Award Nominations
- Conferences 2024
- NTC Conference History
- Newsletter Issues
- Publications
- Distinguished Lecturers 2024
- Distinguished Lecturers 2023
- Past Distinguished Lecturers
- TryNano.org
- Technical Committees
- Technical Committee Award
- Industrial Advisory
- Standards Committee
- Women in Nanotechnology
- Young Professionals
Best PhD Thesis Award
- NTC Publication Awards
- NTC Technical Awards
Join the NTC
Sign up for Mailing List
Visit us on FaceBook
—Latest Publications—
IEEE Transactions on Nanotechnology
IEEE Open Journal of Nanotechnology
IEEE Transactions on NanoBioscience
IEEE Journal of Photovoltaics
IEEE Journal on Exploratory Solid-State Computational Devices and Circuits
IEEE Nanotechnology Magazine
IEEE Nanotechnology e-Newsletter
—-Resources—-
TryNano.org the Nanotech Learning Resource
Latest News
- NANO 2024: Paper Submission Final Deadline
- OJ-NANO CFP: Special Section – IEEE-NSENS 2024: Nano Materials and Devices for Sensors, AI, and Robotics
- OJ-NANO Call for Papers: Special Section – Future Nanocomputing: Trends and Challenges
- Call for Proposals for Future Site for IEEE NMDC 2026
- CFP: IEEE Journal on Exploratory Solid-State Computational Devices and Circuits (JxCDC)
- MARSS 2024: Call for Papers
- NTC WIN to Host a Special Session at NANO 2024
News Categories
Recent comments.
- NTC Chapter’s Innovation Grant - Call for Applications - IEEE Nanotechnology Council on DL Program
- Call for Award Nominations 2023 - IEEE Nanotechnology Council on Awards
- Call for Proposals for Future Sites for IEEE-NANO 2026 and IEEE NMDC 2025 - IEEE Nanotechnology Council on NMDC
- Call for Award Nominations 2023 - IEEE Nanotechnology Council on Call for Award Nominations
- Call for Nominations for 2024 Distinguished Lecturers - IEEE Nanotechnology Council on DL Program
CALL FOR NOMINATIONS for:
Ieee nanotechnology council best phd thesis award in nanotechnology.
(established in 2022)
All nomination materials must reach the Education Awards Committee by March 1 st each year.
Download nomination form.
Description: This annual award recognizes a PhD thesis in nanotechnology with remarkable technology innovation or excellence which should have led to publications in NTC venues including journals and conferences. Any member with no conflict of interest (i.e. advisor-advisee relationship) with any member of the NTC ExCom, NTC Education Committee , or NTC Technical Committees can submit a nomination to the Award Committee for this award. Self-nominations are not allowed. The awardee will be selected by a committee composed of the Chair and 3 members. The Chair is appointed by the NTC President with recommendation from the VP of Educational Activities. The Chair appoints the committee members with approval by the NTC President.
Prize: Total honorarium of $500 and a certificate. Only one allowable recipient selected annually
Eligibility: The author of a nominated thesis must be an IEEE member and NTC participant for at least one year. Only materials from the thesis published in NTC sponsored publications (journals/magazines and NTC financially sponsored conferences) will be considered.
The author of the best PhD thesis in nanotechnology must have graduated within the past 3 years , and not be advised by a voting member of the NTC Executive Committee (ExCom) or a member of the Best PhD Awards Evaluation Committee.
A thesis can be nominated for this award only once. Unsuccessful nominations cannot be carried over or re-submitted over the following years.
There are no other requirements.
Basis for Judging : Each member of the Committee, evaluates each nomination on several aspects, including but not limited to: impact, quality of writing and presentation, relevance of the topic, its influence in nanotechnology based communities.
The range of the score by each member of the committee is from 0-5. The Chair will generate for each nomination a scoring table (with averages) to be distributed to voting members of the Committee. The Committee will rank a final pool of up to 3 nominated papers and recommend the winner. The nominations and recommendations will be presented in a report to the NTC ExCom for voting in its May meeting. The Committee Chair will not vote except to break ties.
Presentation: The award will be presented at the annual IEEE Conference on Nanotechnology (NANO) banquet. Attendance is mandatory to receive the award, but no travel allowance is provided.
- Submission of nominations: Email by March 1 st to the Education Awards Chair, Yu Sun Professor of Mechanical Engineering Canada Research Chair Micro & Nano Engineering Systems Robotics Institute Director University of Toronto, M5S 3G8, Canada Email: sun@mie.utoronto.ca
- Committee’s award recommendation(s): April 1 st
- Notification to awardee(s): May 15 th
For further information, please contact the Awards Committee Chair:
Your Name (required)
Your Email (required)
Subject (required)
Your Message (required)
- Entries feed
- Comments feed
- WordPress.org
- Privacy & Security
- Terms & Conditions
- Nondiscrimination Policy
Advertisement
Nano-biotechnology, an applicable approach for sustainable future
- Review Article
- Published: 09 February 2022
- Volume 12 , article number 65 , ( 2022 )
Cite this article
- Nikta Shahcheraghi ORCID: orcid.org/0000-0002-1748-0050 1 ,
- Hasti Golchin 2 ,
- Zahra Sadri 2 ,
- Yasaman Tabari 3 ,
- Forough Borhanifar 2 &
- Shadi Makani 2
12k Accesses
25 Citations
5 Altmetric
Explore all metrics
Nanotechnology is one of the most emerging fields of research within recent decades and is based upon the exploitation of nano-sized materials (e.g., nanoparticles, nanotubes, nanomembranes, nanowires, nanofibers and so on) in various operational fields. Nanomaterials have multiple advantages, including high stability, target selectivity, and plasticity. Diverse biotic (e.g., Capsid of viruses and algae) and abiotic (e.g., Carbon, silver, gold and etc.) materials can be utilized in the synthesis process of nanomaterials. “Nanobiotechnology” is the combination of nanotechnology and biotechnology disciplines. Nano-based approaches are developed to improve the traditional biotechnological methods and overcome their limitations, such as the side effects caused by conventional therapies. Several studies have reported that nanobiotechnology has remarkably enhanced the efficiency of various techniques, including drug delivery, water and soil remediation, and enzymatic processes. In this review, techniques that benefit the most from nano-biotechnological approaches, are categorized into four major fields: medical, industrial, agricultural, and environmental.
Similar content being viewed by others
Role of Nanomaterials in Environmental Remediation: Recent Advances—A Review
Principles and Potentials of Nanobiotechnology
Nanotechnology and Nanobiotechnology for Environmental Remediation
Avoid common mistakes on your manuscript.
Introduction
The development process of a sustainable future generally consists of methods that ensure the satisfaction of future needs, while fulfills the current generation’s requirements (Raghav et al. 2020 ). To obtain a proper overview of upcoming demands in the future, it is important to anticipate future stressors (e.g., climate change) (Iwaniec et al. 2020 ). Since nanotechnology is applicable in various majors, it is expected that nano-based techniques will take a key role in a sustainable future (Raghav et al. 2020 ), along with making substantial impacts on the universal economic situation due to their wide range of applications in variant industries (Adam and Youssef 2019 ). The unification of diverse fields in science, Inspired by the oneness of nature, is one of the most noticeable subject matters now in the early twenty-first century. Merging four massively operational fields of science has received great attention in recent decades: nanotechnology, biotechnology, information technology, and cognitive sciences (NBIC), which are known as “convergent technologies” (Roco and Bainbridge 2013 ). Non-renewable sources don’t seem efficient for providing large amounts of energy required in various industrial technologies. Convergent technologies are considered as a remedy for this issue. For instance, several nano-based technologies, which consume biological-renewable energy sources, have been introduced (Zhironkin et al. 2019 ). The unification of material on nanoscale makes the mentioned combination of multiple technologies possible. Hence, nanotechnology plays a critical role in NBIC advancement (Roco and Bainbridge 2013 ). According to the definition set by National Nanotechnology Initiatives in 1999, Nanotechnology is an advanced area of research that allows for the production of a wide class of materials in the nanoscale range (less than 100 nm) to make use of size-and structure-dependent properties and phenomena (Luo et al. 2020 ). Although “nano” is defined as that which is less than 100 nm in size, the use of this definition in the biomedical field is less strict and instead may encompass particles up to 1000 nm in size (Landowski et al. 2020 ). Nanotechnology has a wide range of applications, including Agricultural usages (Ndlovu et al. 2020 ), biofuel production (Zahed et al. 2021a ), cancer Immunotherapy (Goracci et al. 2020 ), carbon capture (Zahed et al. 2021b ) and biomarker detection like nanobiochips, nanoelectrodes, or nanobiosensors (Bayda et al. 2020 ). Nanomaterials (NMs) are chemical substances or materials that are manufactured and used at a very small scale, i.e., 1–100 nm in at least one dimension. NMs are categorized according to their dimensionality, morphology, state, and chemical composition (Saleh 2020 ). NMs can be used for rapid extraction of RNA of the novel coronavirus (Kailasa et al. 2021 ). Expanding nanoscience through various branches can eventually enhance the intelligence and capability of individuals, solve various social issues, cure numerous diseases, and generally improve the quality of mankind's life in the long term (Roco and Bainbridge 2013 ). Deploying nanotechnology into biotechnology will help the commercialization process of nano-based techniques and make them more practical in the industry (Maine et al. 2014 ). The idea of developing interdisciplinary research (IDR) (Jang et al. 2018 ) in science presents a promising landscape of the future, in which human intelligence has reached such high levels that the term “superhuman” would be more proper for humankind. According to the Israeli philosopher Harari, with the appearance of a highly technologically advanced society, only individuals with great intelligence and technological advancements can survive through natural selection in society. He states that superhumans will be produced by society eventually, considering the logic of social Darwinism, and this will be a remarkable phenomenon of the twenty-first century (Mantatov et al. 2019 ). One massive application of nanobiotechnology is enhancing the efficiency of various therapies (Table 1 ). The application of nanobiotechnology in delivering chemical drugs or gene modifying agents to their target cells will increase the efficiency of the treatments and reduce the side effects remarkably. Within the previous two decades, RNA-based therapeutic methods, including messenger RNA (mRNA), microRNA, and small interfering RNA (siRNA), have been supremely developed. These therapeutic approaches are expected to be operative in the treatment and prevention of various diseases, such as cancers, genetic disorders, diabetes, inflammatory diseases, and neurodegenerative diseases (Lin et al. 2020 ). In the case of cancers, conventional therapies (surgery, chemotherapy, and irradiation) may cause severe side effects to patients, plus they are often inefficient for disease treatment (Hager et al. 2020 ). Loading anti-cancer drugs into nanomaterials provides a nano-based drug delivery system that detracts the side effects. Platinum (Pt) compounds are one of the most common anti-cancer drugs since 1978. Pt drugs directly aim at the DNA of the targeted cells, thus covering up the defects of the malformed DNA repair mechanisms in cancerous cells. Encapsulating Pt drugs into liposomes constructs a nano-based drug delivery system for treating cancers (Rottenberg et al. 2021 ). Gold nanoparticles (AuNPs) are advantageous options for cancer treatment and diagnosis. AuNPs are created in the size range between 1 and 150 nm and in various shapes, including nanorods (AuNRs), nanocages, nanostars, and nanoshells (AuNSs). AuNPs consist of high rates of biocapability and exhibit controlled patterns of medicine release in the drug delivery process. AuNPs consist of conduction electrons on their surfaces which get excited by certain wavelengths of light. This feature enables AuNPs to adsorb light and produce heat that is fatal to cells. Destroying the cancerous cells with the heat released under irradiation is called photothermal therapy (PTT) or photodynamic therapy (PDT) (D’Acunto et al. 2021 ).
On the other hand, RNA-based therapies can regulate the expression of immune-relevant genes, therefore increasing anti-tumor immune responses directly. Several nanomaterials have been introduced that can deliver nucleic acid therapeutics to tumors and immune cells (Lin et al. 2020 ). There are biomimetic strategies for providing a co-delivery system that is capable of supporting both chemical and RNA-based therapies (Liu et al. 2019 ). Considering RNAs as therapeutic agents or drug targets requires precise knowledge about the 3D structure of specific RNAs. There are reliable algorithms for pronging the second structure of RNAs, but the tertiary architecture which determines the RNA’s functions is quite challenging to anticipate. Bioinformatics provides several methods for predicting the tertiary structures of RNAs such as Vfold, iFoldRNA, 3DRNA, and RNAComposer. They all face particular hurdles, but it should be noted that the field of computational RNA structure anticipation, has a bright future (Biesiada et al. 2016 ). RNA-based vaccines are quite impressive immunotherapeutic tools in cancer therapies. However, the in vivo delivery of synthesized mRNAs could face some obstacles. Encrusting mRNAs with a lipid-polyethylene glycol (lipid-PEG) shell increases the mRNA delivery rate up to 95% more than the conventional nanoparticle-free mRNA vaccines (Islam et al. 2021 ).
In RNA-based nano-techniques, utilizing large-sized RNAs faces several difficulties. Wang et al. have reported an interesting method of using gold nanoparticles (enriched by expanded genetic alphabet transcriptions) to increase the effectiveness of detecting the large natural or artificially synthesized RNAs through an RNA nano-based labeling technique. These techniques are highly dependent on the conjugation between nanoparticles and RNAs (Wang et al. 2020 ). Since gene sequencing is of great importance, multiple biotechnology-based diagnostic tools, including quantitative PCR, DNA barcoding, next-generation sequencing, and imaging techniques are commonly currently used. These methods are considered economically advantageous, along with providing a reliable diagnosis. Incorporating nano-based sensors with mentioned tools increases the sensitivity and spatiotemporal resolution, which are two fundamental features of the gene sequencing process (Kumar et al. 2020 ). Designing nano-based devices for diagnosis of severe acute respiratory syndrome coronavirus 2 (SARS-CoV 2) has been promoted recently. Nanomaterials such as gold nanoparticles, magnetic nanoparticles, and graphene (G) significantly increase the accuracy and decrease the required time and costs. Hence, render beneficial tools for viral detection more effective compared to the traditional techniques. Nanoparticles are specified via anti-bodies to identify particular antigens on the surface of the virus. Suspected samples from the patient, air, and surface can get examined by nano-based serological or molecular diagnosis methods (Abdelhamid and Badr 2021 ).
Nanomaterials can be utilized in the form of membranes. Chemically or physically synthesized nanomembranes remarkably advance the conventional water purification techniques (Lohrasebi and Koslowski 2019 ; Kim et al. 2020 ). Incorporating nanomembranes with bioreactors is the basis of the membrane bioreactor (MBR) technique, which is exploited in wastewater reclamation (Ma et al. 2018 ). Eliminating pollutant components from the environment is one of the main purposes of nanobiotechnology (Table 2 ). In the agricultural fields, nano-bio technologically modified pesticides and fertilizers notably prevent crop loss. Nano-based bioremediation processes have been developed to reduce soil pollutions and are expected to improve both environmental and agricultural approaches (Usman et al. 2020 ). Several studies are expanding the idea of producing nano plants that show better biological performances (e.g., photosynthesis) compared to natural plants (Marchiol 2018 ) (Table 3 ). Enzymes empowered by nanomaterials have rendered higher recovery and productivity rates and thus are potentially able to act spotless in different industrial techniques (Adeel et al. 2018 ; Zhang et al. 2021 ) (Table 4 ).
The objective of this study is to review the applications of nanoscience in enhancing the efficiency of biotechnological methods (Fig. 1 ).
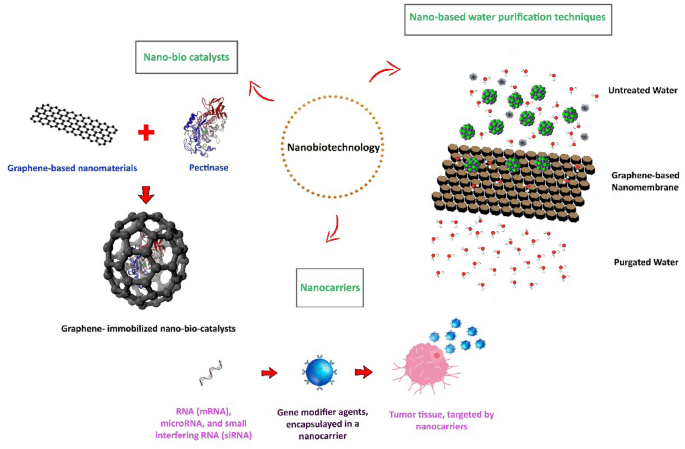
Diverse Applications of Nanobiotechnology: multiple techniques, including Drug delivery-based therapies, remediating processes, and industrial nano-bio catalysts benefit from nano-scaled particles
Application of nano-based materials for drug delivery, therapeutic and diagnostic processes
One recently promoted technique in the gene therapy field is the application of the CRISPR/Cas9 systems, which has been indicated to be highly effective in the treatment of monogenic disorders, non-monogenic disorders, and infectious diseases. Emerging studies have suggested that nanocarriers, which are created from Polymer polyethyleneimine (PEI), are more efficient in delivering CRISPR/Cas9 systems to targeted cells compared to the viral carriers (Deng et al. 2019 ). Gene mutation-related diseases such as cancers and human immunodeficiency viruses are potentially treated by DNA-based vaccines. This type of vaccine enhances disease symptoms by delivering specific gene sequences-which are embedded in plasmids- to targeted cells. Despite having clinical utilization, DNA vaccines face limitations in delivering their genetic cargos to the target cells. Designing efficient nano-delivery systems will eliminate such deficiencies PEI (Lim et al. 2020 ). Virus-like nanoparticles (Jeevanandam et al. 2019 ) seem to form applicable nanocarriers for this purpose (Fig. 2 ).
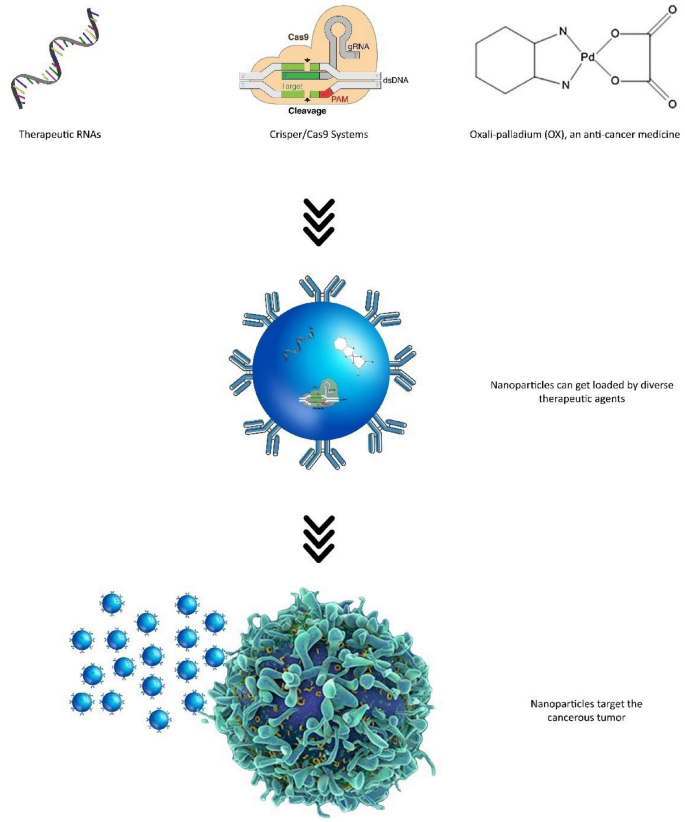
Encapsulating therapeutic agents within nanoparticles: embedding medicine or gene-modifying agents into the nanoparticles remarkably enhances the therapeutic efficiency along with diminishing potential side effects
Nanomaterials used in cancer diagnosis can be mainly divided into contrasting agents (magnetic, iron oxide and gold nanoparticles) and fluorescent agents (quantum dots). Some nanocarriers have inherent optical properties (such as carbon nanotubes, gold and magnetic nanoparticles) that can be converted into high energy to cells for destruction and can serve as nanotheranostics (Barani et al. 2021 ).
Nanomaterials used in smart drug delivery-based cancer therapies are categorized as organic and inorganic materials. Micelles, vesicles, multilamellar liposomes, and solid lipid nanoparticles are some examples of self-assembled organic nanomaterials. Other organic materials are not capable of self-assembling and need to be synthesized, such as nanotubes and dendrimers. Gold nanoparticles, quantum dots, mesoporous silica nanoparticles, and superparamagnetic iron oxide nanoparticles (SPIONs) are classified as inorganic nanomaterials (Lombardo et al. 2019 ). SPIONs are vastly utilized in therapeutic approaches, including cancer therapy, radiation therapy, and tissue engineering. SPIONs are synthesized through different physical, chemical, and biological methods. Bacteria and plants are the biomaterials upon which the biological method is based (Samrot et al. 2020 ). Nanoparticles containing both organic and inorganic materials (hybrid nanoparticles) have been indicated to be highly efficient, as well (Lombardo et al. 2019 ). Embedding targeting ligands (e.g., antibodies, peptides, aptamers, and small molecules) on the surface of nanoparticles assures the delivery of medicines to specific sites in the body, such as tumor tissues. The mentioned process is called: “targeted drug delivery system” (Doroudian et al. 2021 ). There are two types of targeting delivery: passive targeting and active targeting. In the passive form, the high aggregations of medicines at the tumor sites are related to the nano-scaled size of the nanocarriers. The tight junctions between epithelial cells of the vessel tissues prevent the nanoparticles from exiting the vessel. The cancerous cells loosen the tight junctions of the adjacent vessels. Therefore, nanocarriers can pass through the vessel and get into the tumor site. The targeting ligands incorporated with nanoparticles are not responsible for the passive targeting action. The binding between the targeting ligands and the particular receptors on the cancerous cells-which are exclusively found on the surface of the tumor cells- causes a more precise drug delivery, which is known as active targeting (Doroudian et al. 2019 ). Although drug-loaded nanoparticles efficiently carry the medicines to target cites, according to the in-vivo studies, these nanoparticles might not be quite biodegradable. Hence using such nanoparticles could lead to toxicities and side effects. It is worth mentioning that Zhou et al. have developed biodegradable nanoparticles using poly (aspartic acid) (PASP) microtube, a thin Fe intermediate layer, and a core of Zn (Zhou et al. 2019 ).
Nano-based drug delivery systems provide highly promising prospects for treating neurodegenerative disorders. It is reasonable to assume that treating neurological diseases by conventional drug delivery systems is extremely challenging due to the presence of the blood–brain barrier (BBB). The blood–brain barrier prevents the entrance of therapeutical agents to the central nervous system (CNS), therefore, making the conventional therapies inadequate. The blood–brain barrier provides a stable environment for the CNS and regulates the cell-to-cell interactions, which take place in the CNS. The dysfunction of the blood–brain barrier leads to severe neurodegenerative disorders (e.g., Parkinson’s disease (PD), Alzheimer’s disease (AD), amyotrophic lateral sclerosis (ALS), and multiple sclerosis (MS)). The blood–brain barrier is responsible for the proper functioning of the CNS, so naturally, it has a super-sensitive permeability. This feature of the blood–brain barrier is highly related to the tight junctions between the barrier’s cells. Only 1–4 percent of most CNS medicines succeed in passing the blood–brain barrier. Nanoparticles are more likely to pass the barrier because of their nano-scaled size. Encapsulating drugs in nanoparticles can significantly increase the drug transmission rate through the blood–brain barrier (Furtado et al. 2018 ). For instance, graphene, metals, carbon-nanotubes, and metal-oxides are the nanomaterials that can get exploited in the treatment procedure of patients with Alzheimer’s disease (AD). AD is caused by different genetic and environmental cues. Chemical and electrical malformations are observed in the brain of an AD patient. Acrine and physostigmine, which are conventional medicines for AD, have been proved to stimuli severe effects on the gastrointestinal tract and nervous system. Therefore, attention is drawn to nano-based therapies (Nawaz et al. 2021 ). Marcos-Contreras et al. have proposed that the augmentation of VCAM-1 ligands to the drug-loaded nanocarriers can significantly improve the cerebral accumulation rate of nanoparticles in inflamed brains (Marcos-Contreras et al. 2020 ) (Fig. 3 ).
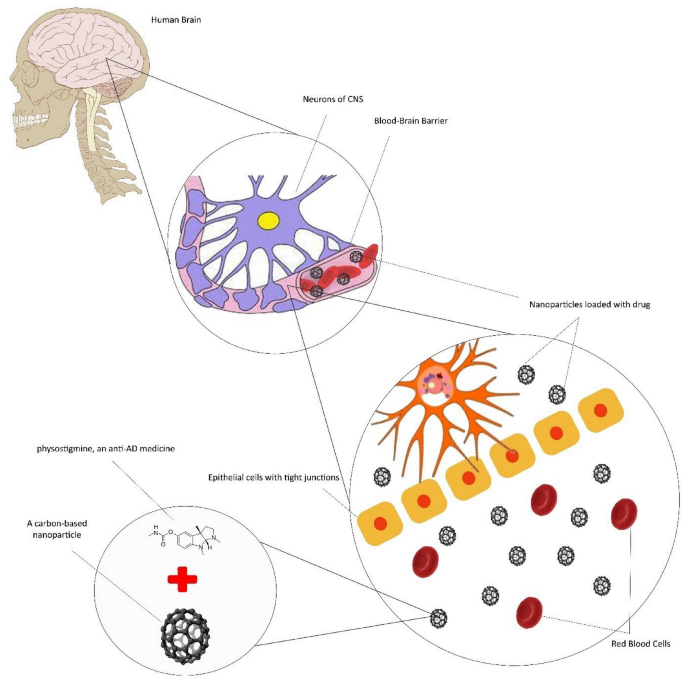
Nano-based drug delivery in the therapies of neurodegenerative disorders: blood–brain barrier (BBB) is a noticeable obstacle for conventional medicines; however, drugs encapsulated within nanoparticles efficiently penetrate through the BBB and reach the central nervous system (CNS)
Although nano-based medications of neurodegenerative disorders seem spotless theoretically, the internal environment of the body puts out several obstacles on the path of the medicine nano-delivering. For instance, lipid nanoparticles (LNPs) may safely carry their therapeutic cargos to the targeted cells, but if the drug needs to reach the cytoplasm, lipid nanoparticles are not capable of efficiently crossing the cell membrane. Small interfering RNAs (siRNAs) are delivered to hepatocytes via lipid nanoparticles, but only 2% of them accomplish reaching to the cytoplasm. It should be mentioned that big data and computational methods can help scientists to predict the in-vivo challenges of nano-drug delivery to design proper techniques to overcome them (Paunovska et al. 2019 ). Besides, bioinformatics provides tools for measuring the interaction rate between exploited nanomaterials and drug targets (Nawaz et al. 2021 ). Designing efficient nanomaterials is fundamental for nanotechnological approaches. Carbon nanotubes (CNTs) and graphene-based nanomaterials have been vastly utilized in nanotechnology during the last two decades (Kinloch et al. 2018 ). As a case in point, Single-walled carbon nanotubes (SWCNTs) are considered as excellent options for designing nano-based biomedical approaches, including but not limited to drug delivery systems. The most noticeable features of SWCNTs are their great photophysical properties (Farrera et al. 2017 ). Even though Carbon nanotubes (CNTs) and graphene-based nanomaterials have unique qualities such as high flexibility, they face some challenges in their load transfer capability, dispersion, and viscosity. Hence, creating more applicable and eco-friendly nanomaterials has drawn intense attention (Kinloch et al. 2018 ). AlNadhari et al. have introduced algae as a green and eco-friendly source of materials that can be used in nanoparticles. Algae-based nanoparticles in the biomedical field consist of therapeutical characteristics, such as antibacterial, anti-fungal, and anti-cancer features (AlNadhari et al. 2021 ). Milk-derived proteins such as β-lactoglobulin (β-LG), lactoferrin (LF), and the caseins (CN) are other biological alternatives for synthesizing nanocarriers. Anti-cancer medicines have been embedded into protein-based nanocarriers and successfully deteriorated cancerous tumors (Tavakoli et al. 2021 ). Azarakhsh et al. have demonstrated specific binding sites for the anti-cancer drug, Oxali-palladium (OX) and iron nanoparticles (NP) on the Beta-Casein (β-CN). Hence, the Beta-Casein can perform as an efficient carrier for both agents (Azarakhsh et al. 2021 ). One common strategy in designing nanocarriers for cancer therapies is to create nanoparticles that can detect the vitamin or growth factor receptors on target cells. Cancerous cells usually over-express the receptors for such nutrients so that they can keep their high proliferation rate (Peer et al. 2020 ). Reprogramming the nutrient signaling and micropinocytosis of the cancer cells seriously affects the efficacy of Nano-particulate albumin-bound paclitaxel (nab-paclitaxel, nab-PTX); which is one of the most commonly prescribed nanomedicines (Li et al. 2021 ).
Antimicrobial peptides (AMPs) are short-chain, often cationic, peptides possessing several attributes which make them attractive alternatives to conventional antibiotics with s a low likelihood of resistance developing in target organisms (Meikle et al. 2021 ). Conjugation and functionalization of nanoparticles with potentially active antimicrobial peptides has added advantages that widen their applications in the field of drug discovery as well as a delivery system, including imaging and diagnostics (Mohid and Bhunia 2021 ).
Silver nanoparticles coated with zinc oxide (Ag@ZnO), can stimulate proliferation and migration of human keratinocytes, HaCaT, with increased expression of Ki67 and vinculin at the leading edge of wounds. Interestingly, Ag@ZnO stimulates keratinocytes to produce the antimicrobial peptides hBD2 and RNase7, promoting antibacterial activity against both extracellular and intracellular Staphylococcus aureus isolated from wounds (Majhi et al. 2021 ).
Wound dressing is an important action against an injury. In recent years, nanotechnology has been combined with wound dressing techniques, and there are several new materials and techniques available for this action. The nanoparticles’ dimensions make them suitable for penetrating into the wound. Thus, bioactive agents and drugs can be released locally (De Luca et al. 2021 ). Numerous synthetic and natural materials have been applied for wound healing; Hyaluronic Acid, as an illustration, is one of the most-used materials (Ahire and Dicks 2016 ).
In 2017 Polyethylene Oxide (PEO)-hyaluronic acid (HA) nanofibers as an inhibitor of Listeria monocytogenes infection (Ahire et al. 2017a ). Gauze is a traditional wound dressing used to protect dermal wounds from bacterial infection. In a study in 2021, an antibacterial gauze was prepared by the combined use of antimicrobial peptides and AgNPs. The prepared antibacterial gauze showed excellent antibacterial activity against E.coli, S. enteritidis, S. aureus , and B. cereus and also exhibited good biocompatibility (Chen et al. 2021a , b ). In 2014, Ahire and Dicks introduced 2,3-Dihydroxybenzoic Acid-Containing Nanofiber as a suitable nanomaterial for wound dressing as it prevents Pseudomonas aeruginosa infection (Ahire and Dicks 2014 ). To inhibit the growth of this microorganism, Copper-Containing Anti-Biofilm Nanofiber Scaffolds can be used too. Copper-containing nanoparticles have the potential of inhibiting Escherichia coli growth either (Ahire et al. 2016 ). Surfactin-loaded nanofibers are also a great candidate to be used in wound dressings or in the coating of prosthetic devices to prevent biofilm formation and secondary infections (Ahire et al. 2017b ). In addition to nano-therapies, nano-diagnostic agents- metal nanoparticles- have been indicated to be highly applicable in the detection of viruses, including covid-19 (Fouad 2021 ). Several biotic [e.g., algae (AlNadhari et al. 2021 ) and viral capsid (Jeevanandam et al. 2019 )] and abiotic [e.g., gold, silver, graphene oxide, and zin oxide (Fouad 2021 )] nanomaterials have been reported to be applicable in biomedical processes. The combination of biotic and abiotic sources provides efficient nanomaterials as well. For example, the highly effective graphene-starch nanocomposites, are resulted from embedding graphene-based nanomaterials into the starch biopolymers (Mishra and Manral 2021 ). The delivery of therapeutics via nanoemulsions (NE) has shown striking results. Sánchez-Rubio et al. have successfully defeated deficiencies of vitamin E (e.g., hydrophobicity and low stability) by creating nanoemulsions comprising vitamin E. the sperm samples derived from the red deer’s epididymal tissue was treated with the mentioned nanoemulsions and the sperms’ viability and resistance against oxidative stress, was increased (Sánchez-Rubio et al., 2020 ). Jeong et al. have reported another growth-promoting method that elevates the maturation process of cultured cells. The mentioned technique aims to develop an extremely operational and cost-effective bioreactor that enables in-vitro maturation of heart tissue. Next-generation stage-top incubator (STI) containing nano grooves patterned PDMS diaphragm (NGPPD) was designed to boost cell maturation and myogenic differentiation. The surface of NGPPD was covered with a slim layer of gold (Au) (Jeong et al. 2021 ). Microfluidic systems are proven to have applications in biological analysis, tissue engineering, etc. Embedding nanolitre volumes into micro-sized fluidic channels is the basis of the aforementioned technique (Valencia et al. 2020 ).
Application of nanoparticles on bioreactors as contributory agents
Since wastewater reclamation is a universal challenge and plays a major role in providing clean water for many people across the world, various techniques have been developed for this purpose. Among them, the application of membrane bioreactors (MBRs) in water purification has attracted great attention recently. In the MBR technique, the conventional activated sludge (CAS) process is incorporated with a filtration process provided by a physicochemical membrane (Ma et al. 2018 ). It has been shown that treating the mentioned membrane with nanoparticles in different types of MBR techniques can significantly improve the efficiency of the process (Abass and Zhang 2020 ; Jiang et al. 2019 ). The pharmaceutical industry produces one of the most pollutant wastewaters; which contains various amounts of organic compounds, including benzene, polynuclear aromatic hydrocarbons (PAHs), and heterocyclic, etc. these compounds have high Chemical Oxygen Demand (COD) and low degradability; which makes conventional biological treatments inefficient for treating them. However, applying O 3 , O 3 /Fe 2+ , O 3 /nZVI (nano zerovalent iron) processes in wastewater purgation has made noticeable signs of progress. Nano catalytic ozonation process (O 3 /nZVI) in a semi-batch reactor has the highest effect on advancing degradation amongst all (Malik et al. 2019 ). An experiment conducted in southern Tehran succeeded in removing the Methyl Tertio Butyl Ether (MTBE) and benzene from groundwater, using Fenton’s chemical oxidation with stabilized nano zerovalent iron particles (S-NZVI) as a catalyst. The removal efficiency of MTBE and benzene were increased to 90% and 96%, respectively, by reducing the pH of the reaction environment down to 3.2. Acidification of the environment decreased iron consumption as well (Beryani et al. 2017 ).
Nano-bioremediation
One green and cost-effective approach for treating the pollutant soils to reduce their toxicity is applying living organisms (bacteria, fungi, plants, etc.) through a process named: “bioremediation.” Integrating bioremediation with nanoparticles increases the efficiency of the process (Usman et al. 2020 ). The technology of nano-remediation is a sustainable method to reduce the contaminants of the soil by various means (Yue et al. 2021 ; Sajjadi et al. 2021 ; Lian et al. 2021 ). As an example, the reduction of Cr (VI) levels using this technology is known to be worthwhile in many aspects (Azeez et al. 2020 ; He et al. 2020 ). Chemically active nanoparticles can trigger the dechlorination/dehalogenation process in organic pollutants and neutralize them, consequently. Even the toughest pollutants are targeted in this nano-bio-based remediation method. The time needed for the purgation of highly contaminated soils will be minimized by virtue of the mentioned technique (Usman et al. 2020 ). Iron oxide nanoparticles (NP) and Fe 3 O 4 /biochar nanocomposites are vastly exploited in the synthesis of nanoparticles of nano-bioremediation (Patra Shahi et al. 2021 ). It is worth noting that nano zerovalent iron (nZVI) is an effective technology in the case of remediation that has been applied broadly in recent years due to high levels of reactivity for contaminants (Luo et al. 2021 ; Visentin et al. 2020 ; Ken and Sinha 2020 ; Hou et al. 2019 ; Zhu et al. 2019 ).
The bioremediation process can be used in water purification as well. Separating solid components from liquid waste is a necessary stage in the water remediation process. The fresh market waste may contain infectious components, which can seriously harm humans and plants. Hence, it is important to develop methods to collect, separate, and treat these adverse agents. Solid wastes in the wastewater contain high amounts of carbohydrates and proteins, and they provide matrices for the colonization of infectious organisms. Altogether, the presence of solid wastes improves the growth rate of pathogenic organisms. After solid matters got collected, they should be stored and treated immediately. The treatment process must not be delayed because the enriched environment of the solid wastes can easily get corrupted. One way to treat them is through triggering the fermentation and composting processes. Adding effective microorganisms (EM), such as lactic acid/phototropic bacteria and yeast, accelerates the conventional fermentation and composting processes used for the solid waste treatment (Al-Gheethi et al. 2020 ). Costa et al. have sequenced the whole genome of the strain Streptomyces sp. Z38, and detected growth-promoting, heavy metal-eliminating, and anti-microbial features within specific biosynthetic genes. Streptomyces sp. Z38 seems to be a suitable agent for bioremediation due to its ability to decompose heavy metals such as Cr (VI) and Cd (II). Costa et al. have supplemented the bioactive water (BW) extracted from Streptomyces sp. Z38 with AgNO 3 additives and produced silver nanoparticles (AgNPs) that are capable of performing the bioremediation process (Costa et al. 2020 ). There are other effective nanomaterials exploited to reduce many pollutants from soil and wastewater. For instance, utilization of nano-manganese oxide to eliminate ZnII/CoII from water (Mahmoud et al. 2020 ), application of nano-semiconductors on water and their Photocatalytic effectiveness (Oliveira et al. 2021 ), nano-scaled Iron (II) sulfide exploited to reduce hexavalent chromium from soil (Tan et al. 2020 ), production of nanocomposite for eliminating viruses (Al-Attabi et al. 2019 ), and successful application of nano biosurfactants which cause no toxicity for the environment (Debnath et al. 2021 ). Nano-bioremediation as an emergent approach causes some concerns and benefits at the same time. It is possible that nanomaterials exploited in this method would be a threat to the organism populations that exist naturally in water bodies. On the other hand, new living organisms would be introduced through bioremediation. The mentioned two scenarios can potentially put the anthropogenic features of ecosystems in danger (Weijie et al. 2020 ). Concerning this problem, however, scientists are trying to apply new methods to remove nanoparticles from marine ecosystems via other technologies (Ebrahimbabaie et al. 2020 ).
Designing nano-based water purification techniques, to overcome the problem of lack of clean water, across the world
Waterborne diseases that cause almost 10–20 million deaths annually are considered crucial health-related issues. According to the World Health Organization and environmental protection agencies, the pollution level of several water bodies has long crossed the defined limitations. Thus, developing methods for purging water from adverse components is of great concern (Sahu et al. 2021 ). The water purification process profits extremely from nanobiotechnology. Nanoparticles are extremely efficient in eliminating pollutants (e.g., dye components) due to their nano-scaled size and increased surface areas. In the case of dye removal, magnetic nanoparticles have been proved to be proper candidates (Lohrasebi and Koslowski 2019 ). Nanoadsorbents such as silica gel, activated alumina, clays, limestone, chitosan, activated carbon, and zeolite are cost-effective and profitable options for eliminating the contaminating agents during water purification process (Ali et al. 2020 ).
Copper and copper compounds are potent biocides and have been utilized as a disinfectant for centuries due to their anti-microbial properties. It becomes more functional in its nano form and exhibits outstanding synergist, anti-fungal, and anti-bacterial effects (Bashir et al. 2021 ).
Copper nanoparticles have the potential of combination with other materials like Polyacrylonitrile (PAN) nanofibres and Polyethylene Terephthalate Filters to act more beneficial (Ahire and Neveling 2018 ; Nguyen et al. 2021 ).
Metallic nanomaterials, carbon-based nanomaterials, nanocomposites, and dendrimers are four major types of nanomaterials that can be applied in wastewater purgation (Murshid et al. 2021 ). Graphene-based nano-channels, which are inspired by aquaporin channels, have been utilized as water filters and are expected to enhance the water permeability and the salt rejection rate. It is worth noting that the efficiency of these filters can be affected by various factors. For example, it has been indicated that increasing the charges on the channel will decrease the water flow through the channel but, on the other hand, increase the ion rejection rate (Lohrasebi and Koslowski 2019 ). Carbon nanotubes (CNTs) have rendered noticeable results in eliminating the water contaminants, as well (Kutara et al. 2016 ).
The biosafety of water purification via finger-sized unit (FSU) has been certified by cellular and animal tests. In one study, Li et al. loaded 3D printed finger-sized units with prepared wheat straw (WS). To prepare WS for mentioned technique, the carbonized wheat straw (CWS) was adjusted with nano-scaled zinc oxide during an in-situ surface-modification process (CWS/ZnO). The resulted FSU was able to reduce bacteria, organic dyes, and heavy metal ions; therefore, elevating the purification efficiency. Since WS is one of the major agricultural wastes worldwide, applying it in water purification will not only cost very low but will reduce the air pollution which is caused by burning WS in many countries. The WS has a hallow, flexible, and electrical conductor structure. These features make WS a great candidate for enhancing water purification performance (Li et al. 2019 ).
For designing a nano-based filtering membrane, nanoparticles don’t always have to be chemically synthesized or externally applied on the membrane. An emerging study has suggested a top-down approach that uses biomass to provide a functional membrane for the purification of the emulsions. This method can be used massively in cleaning oily waters resulting from industrial or domestic activities. The biomass used in the mentioned technique is wood tissue. The lignin and hemicellulose fractions are removed sectionally, and therefore, a highly porous, flexible, and durable membrane is provided. Since the lignin is removed and there is no hydrophobia left, the resulting wood membrane consists of outstanding water-absorbing and anti-oil properties. The wood-nanotechnology-based membrane shows significant efficiency due to its numerous advantages, including being green, economical, easy to produce, durable, and having selective wettability (Kim et al. 2020 ).
Rezaei et al. have synthesized a flower-shaped ZnO/GO/Fe 3 O 4 ternary nanocomposite through the co-precipitation method, which is considered a rather fast and easy synthesis approach. The mentioned nanocomposite improves the ZnO degradation through a performance with an efficiency that is more than two times greater than the efficiency of the methods using ZnO particles alone. Hence, the ZnO/GO/Fe 3 O 4 ternary nanocomposite seems to be an economical and time-saving approach for wastewater remediation (Rezaei et al. 2021 ).
It is worth noting that the vast uses of nanoparticles in different industrial products increase the risk of the inevitable release of nanoparticles into the environment, and therefore cause some concerns about the potential damages of nanobiotechnology. The urban wastewater seems to be highly exposed to industrial nanoparticles. The high concentrations of nanoparticles in the urban wastewater contaminate the sewage sludge, consequently. Wastewater treatment plants (WWTPs) are currently exploited to remove nanoparticles from wastewater and sewage sludge (Wang and Chen 2016 ). Nanoparticles synthesized and utilized in the industry can end up in marine ecosystems. Nanoparticles are developed from various chemical components such as carbon, silver, gold, and copper, which are potentially hazardous to live organisms. Since nanoparticles are extremely small in size, likely, they will easily enter the bodies of aquatic animals. It has been demonstrated that the accumulation of nanoparticles in the animal’s body can cause severe morphological and behavioral deformities. Genetic materials of cells may undergo various changes as well (Gökçe 2021 ).
FeO ion, which is known as Nanoscale zerovalent iron particles (nZVI), is massively used in the synthesis of nanoparticles applied in wastewater nano-based treatments. Bensaida et al. have shown that combining nZVI with another metal (Cu) enhances the growth of the microbial populations in the wastewater treated with this nZVI\Cu bimetallic nanoparticles (Bensaida et al. 2021 ).
Exploiting nanobiotechnology-based methods in food industry
Nanotechnology-based pharmaceuticals were developed primarily, but wide applications of nanoscience in food and agricultural industries have been introduced as well (Sahani and Sharma 2020 ). Utilizing nanoscience in any stage of the food production process-either cultivation, production, post-harvest processing, or packaging—seems to be lucrative. The application of nano-based methods in the food industry has various advantages, but the most arguable of them would be its impact on shelf life augmentation and spoilage prevention (Bhuyan et al. 2019 ). Since Oxygen is known as an important cause of food spoilage in the food industry, scientists have developed the technology of advanced coatings based on nanotechnology to prevent Oxygen from spoiling the product (Rovera et al. 2020 ). Multiple nanoparticles have the potential to deliver nutritional or antimicrobial components into food materials (Bhuyan et al. 2019 ). It has been reported that nanotechnology is a good option to deliver pesticides and nutrients successfully into the soil and improve the strength and tolerance of products in different stressful situations and reduce the probable contaminations (Ali et al. 2021 ). Among different nanoparticles such as silver, titanium dioxide, and zinc oxide, nanoliposomes are found to be small and have a large surface area which makes them more adhesive to biological tissues- therefore more bioavailable in comparison to others. Nanoliposomes are suitable candidates for creating a delivery system during food preparation. Food provided with the help of nanotechnology is called “Nano food” (Bhuyan et al. 2019 ). Nano foods can perform as therapeutic options. It is interesting to mention a recent study that has proposed exploiting nanoemulsions to convey needed nutrients to gastrectomy patients. These types of patients usually suffer from conditions like anorexia, energy deficit, and malnutrition, which can be treated by efficient nutrition delivery provided by nano food (Razavi et al. 2020 ). As mentioned earlier, in the food preparation process, antimicrobial components can be delivered along with nutritional components via a nano-based delivery system. Polyphenols are great examples of substantial antioxidant and antimicrobial agents in the food industry. Nevertheless, polyphenols have some limitations, including instability, low solubility, inefficient bioavailability, and being drastically susceptible to being degraded. There are several factors that reinforce degradation: Oxygen, light, pH, and interactions between polyphenols and other components in food. Polyphenol-loaded nanoparticles relatively overcome the mentioned obstacles due to their capacity to protect phenolic compounds against degrading processes (Milinčić et al. 2019 ). As a renewable and biodegradable source, starch is a useful polymer that has been applied in different fields such as the pharmaceutical and food industries. Nano-size starch is an advanced material with new abilities in the matter of hydrophobicity and stability (Wang and Zhang 2020 ). In the field of the food industry, there are also many other new methods based on nanotechnology, for instance, designing natural proteins as nano-architectures to deliver nutraceuticals (Tang 2021 ), new strategies for packaging food products by exploitation of the knowledge of nano-biotechnology, and nanomaterials (Reshmy et al. 2021 ; Jogee et al. 2021 ; Tiwari et al. 2021 ), utilization of the nano-delivery techniques to overcome the problems of consuming bioactive ingredients (Hosseini et al. 2021 ; Ozogul et al. 2021 ), producing nanoparticles in the shape of powder using the nanospray driers (Jafari et al. 2021 ), detection of food contaminants by nano-Ag combinations (Yao et al. 2021 ), and even the application of nano-engineering in the field of the beverage industry (Saari and Chua 2020 ).
Nano-bio catalysts; an attempt to remove the barriers of enzymatic bioprocesses in the biotechnology industry
Organic enzymes, which are normally found in nature, have large applications in the biotechnology industry. Since organic enzymes are green and eco-friendly, they are usually preferred to commercially synthesized enzymes. Pectinase is considered to be extremely useful for manufacturing purposes. Pectinase application in industrial bioprocesses covers a large range from clarification of juice/ wine and tea/coffee fermentation to wastewater and industrial waste remediation. All enzymes- regardless of being organic or chemically synthesized- consist of limitations that make their usage challenging. Three major disadvantages of enzymes are inefficient recoverability, operational stability, and recyclability (Zhang et al. 2021 ). Functional nanomaterial-based bio-carriers render a proper environment for the enzymatic immobilization process, therefore facilitating recovery and recycling of enzymes and enhancing the efficiency of bioprocesses in the long run. Accordingly, designing nano-based carriers with these features has been attracted great attention. To achieve this aim, Graphene- immobilized nano-bio-catalysts have been proved to be greatly useful due to the Graphene’s characteristics: electrical, optical, thermal, and mechanical high potency (Adeel et al. 2018 ; Zhang et al. 2021 ).
Nanomaterial-based nanocatalysts are useful in optimizing the biodiesel production process. This ability is related to the features of nano-scaled materials, including crystallisability, high adsorption and storage potential, having catalytic activities, and great stability and durability. Various materials can be used to create nanoparticles for this mean; some examples are metal oxide (calcium, magnesium oxide, and strontium oxide), Magnetic material, and Carbon. Carbon-based nanomaterials consist of multiple types, such as carbon nanotubes, carbon nanofibers, graphene oxide, and biochar.
All examples mentioned above have been proved to be highly effective in increasing the efficiency of the biodiesel synthesizing process and reducing the time and cost required for operating the process without utilizing nanotechnology (Nizami and Rehan 2018 ).
Replacing non-renewable energy sources with renewable ones is a great step in guaranteeing a sustainable future. Various devices, including solar and fuel cells, have been developed for this purpose. Conventional fuel cells are made from metal reactants instead of fossil fuels. They provide an electron circulation, transfer electrons from the substrate to specific electrodes, and eventually produce sustainable energy. The metals used as catalysts in fuel cells (e.g., hydrogen, methane, and methanol) are usually expensive and non-durable. On the other hand, biofuel cells use cost-effective bio-catalysts (e.g., microbes and enzymes) instead of metal catalysts. Despite the mentioned advantages, biofuel cells have one major limitation: the low rate of electron transfer between substrate and electrodes, which is significantly enhanced by supplementing biofuel cells with nanomaterials. Nanomaterials are able to assemble the substrate (e.g., enzymes) with the electrodes. In other words, using them in the structure of electrodes, the electron absorption of electrodes improves- related to the high surface area rate of nanomaterials- therefore, a direct transition of electrons between enzymes and electrodes develops. Silver nanoparticles-Graphene oxide (Ag-GO), Graphite, Carbon-nanotube forest (CNTF), Carbon nanotube (CNT), and Nitrogen-doped hollow nanospheres with large pores (pNHCSs) are the nanomaterials applied in nano- biofuel cells. Respectively, Glucose oxidase (GO x ), Glucose oxidase and Laccase, Fructose dehydrogenase & laccase, Glucose oxidase and laccase, and NADH dehydrogenase form the enzymatic system of each nanomaterial (Sharma et al. 2021 ).
Metal–organic frameworks (MOFs); highly advantageous materials
Porous materials are known to be highly advantageous due to their high absorption and surface areas. Zeolites, activated carbons, and silicas are examples of this family, but the most eminent member among them are Metal–organic frameworks (MOFs). MOFs have features that make them unique for several applications. For example, MOFs show a high absorption rate, which is caused by their high surface areas. Another property of MOFs is their possession of several adjustable microporous channels, which makes it easy to produce different and changeable functional sites through them. The latest feature brings MOFs the shape and size selectivity. By controlling the starting materials and reaction parameters, it is possible to determine the morphology of MOFs (Kinik et al. 2020 ; Jun et al. 2020 ) into various shapes, including granule, pellet, thin-film, gel, foam, paper sheet, monolith, and hollow structures (Kinik et al. 2020 ).
There are two types of MOFs: (1) neutral MOFs and (2) ionic MOFs. Ionic MOFs are able to be used directly in anion purgation processes. For example, one approach for reducing the pollutant anions from the environment is synthesizing a cationic framework along with extra-framework anions. The synthesis of mentioned frameworks occurs by utilizing neutral nitrogen donors. The extra-framework anions will exchange with pollutant anions through an Ion exchange process called: “Anion trapping”.
Anions are extremely abundant in nature. One of the most pollutant and hazardous anions is phosphates. These toxic anions are highly used in pesticides. Other examples of toxic anions, which are considerably frequent in industrial wastes, are the bulky anions. These are the dye molecules exploited in industry. Various diseases like cancers, lung/kidney dysfunction, and brain diseases, including Alzheimer’s, are caused by dangerous anions like those mentioned above. Hence, creating methods that are able to recognize and delete the perilous anions from the environment is one of the most appreciated scientific approaches. MOFs have been proved to be functional for this mean (Desai et al. 2019 ).
Since MOFs have considerable surface areas and modifiable structure—different open metal sites and other functional groups can be introduced into their frameworks—they are suitable options for numerous applications which are generally related to detection and storage. In the case of storage, they exhibit acceptable physical adsorption for CO 2 (one of the major causes of global warming), H 2 (a clean energy source), and Methane (CH 4 ). The ability to adsorb variant components makes MOFs proper for water purification applications. Several toxic and harmful components which are responsible for water contamination, including organic pollutants (like dyes and oils) and heavy metal ions, can be detected, adsorbed, and removed by MOFs. Introducing different chemical groups into MOFs creates different internal interactions, which enable MOFs to detect target molecules functionally. Therefore, they can be used in active centers of catalysts, photocatalysts, and biosensors (Kinik et al. 2020 ).
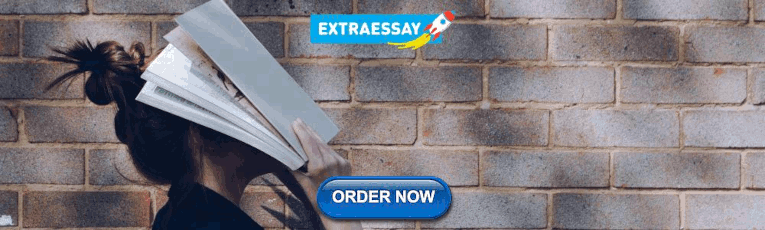
MOFs-based nanozymes
Nanozymes are classified into two types: (1) natural enzymes that are incorporated with nanomaterials and (2) nanomaterials that exhibit inherent enzymatic features. Exploiting MOFs as nanomaterials in nanozyme structures will produce an emergent form of nanozymes, called: “MOF-based nanozymes”; which have multiple advantages over conventional forms. MOFs provide more catalytic sites, simplify the entrance of small substrate molecules -due to their porous structure-, enhance the substrate exclusivity, and altogether improve the catalytic function of enzymes. MOF-based nanozymes are effective in designing biosensors, biocatalysis, and biomedical imaging techniques. A recent promising application of them is in cancer therapy which reduces side effects significantly (Ding et al. 2020 ).
Agricultural usages of nanobiotechnology
Applying nanobiotechnology in agriculture to improve the agricultural production rate has been of great importance recently. Achieving this purpose will solve several problems related to the universal hunger dilemma. Several nanofertilizers, nano pesticides, and nano-bio sensors have been created, which are able to increase crop value and decrease crop loss caused by agricultural pests (Usman et al. 2020 ). Conventional chemical pesticides and fertilizers can be deteriorative for soil composition and fertility. This happens because chemical residues can target many molecules other than the ones that have been defined as their main targets (Chhipa 2019 ). Besides, pesticides can have ruinous impacts on the microorganisms that naturally exist in the environment and are required for the crop’s growth (Nehra et al. 2021 ). Utilizing nanoparticles can considerably reduce such unwanted events due to the high exclusivity of these particles. Silver, zinc, iron, titanium, phosphorus, molybdenum, and polymer are suitable materials to be used in the structure of agricultural nanoparticles (Chhipa 2019 ). Nanoparticles containing nutrients, fertilizers, and pesticides, can be sprayed externally to the plant. The folium will adsorb the nanoparticles and send them to the soil (Chugh et al. 2021 ).
Another application of nanobiotechnology in diminishing the damages of some traditional pesticides is designing nano-bio sensors that can efficiently detect toxic pesticides. Dichlorvos is one of these toxic pesticides that accumulate in the air, soil, water, and crops; and therefore causes neural, genetical, respirational, and muscular disorders. Dichlorvos-sensitive Nano-biosensors comprise immobilized enzymes embedded in nanomaterials. Acetylcholinesterase (AChE), tyrosinase enzymes, and some others are options for the enzymatic part of the nanodevice. For the nano- matrix section, both organic (carbon, graphene, chitosan, and onion membrane) and inorganic (silver, gold, silica, and Titania) options are available (Mishra et al. 2021 ). Nanomaterials can enhance the remediation process of contaminated soils through distinct abiotic and biotic directions, including the nano-bioremediation process (Usman et al. 2020 ).
Other than improving the functions of existed plants, the possibility of introducing engineered plants with better performances has been discussed recently. The term “plant nano bionics” refers to a pioneering idea of involving nanoparticles in living plants to make their intrinsic functions adjustable. The landscape of this idea is designing engineered artificial photosynthetic systems, enhancing the growth rate of this new type of plant, and many other novel applications which are expected to grow extremely in the years ahead (Marchiol 2018 ).
It is necessary to mention that inorganic nanoparticles that may be found in consumer products, may alter the gut composition and could lead to various gut-related diseases. Thus, there have to be some limitations in nanoparticle agricultural usages (Gangadoo et al. 2021 ; Ghebretatios et al. 2021 ).
Using nanoparticles in cosmetic products
Nowadays, due to special and distinctive physicochemical characteristics, nanomaterials are being vastly used in different industries. Recent studies are focused on applying nano-based technologies to improve the quality of cosmetic products. Nanostructures are about to deliver active ingredients to the skin. For this reason, it is more suitable to use lipid particles that are better adaptable to dermal absorption. The high stability of the combination of nanomaterials and lipid particles with cosmetic components indicates high efficiency. However, the probable risks of this method should not be ignored (Benrabah et al. 2020 ; Khezri et al. 2018 ). Producing nanoparticles using plants (Phyto-metal nano-based particles) is another advantageous method to decrease the toxicity of nanomaterials and their hazardous effects on the body. For this reason, this material is suitable for dermal uses and cosmetic applications (Paiva-Santos et al. 2021 ). Chitosan nanoparticles with better penetrability (Ta et al. 2021 ; Sakulwech et al. 2018 ), Gold and silver nanoparticles with a higher ability to reduce microbial contaminants (Séby 2021 ), Titanium dioxide (TiO 2 ) nanoparticles deposited with yttrium oxide (Y 2 O 3 ) with better attenuation of ultraviolet radiation and less cytotoxicity (Borrás et al. 2020 ), nanoparticles with high uptake of oily components (de Azevedo Stavale et al. 2019 ) are other examples of the efficient application of nanotechnology in the field of cosmetic products.
Since nanoparticles are small in size, they exhibit perfect penetrability through the skin. Hence, using nanoparticles in cosmetic productions improves the supplementation of skin, hair, or teeth with active cosmetic ingredients (APIs). It is important to note that utilizing nanoparticles for several applications, as an emerging field of science, causes various concerns about being toxic or harmful for the body or the environment. The cosmetic industry’s products are commonly designed for skin, hair, nail, teeth, and therefore, are directly related to the health of the human body. Thus, it is reasonable to assume that there are even more concerns about using nanoparticles in this industry compared to others (Santos et al. 2019 ).
In addition to these cases, nanotechnology can be useful for the detection of harmful components in cosmetic ingredients. Therefore the application of methods like covered iron oxide nanoparticles with silver for detection of mercury contamination in cosmetics (Chen et al. 2021a , b ), Quantitative assessment of the Triamcinolone acetonide (TCA) (which is a hazardous component in high doses) using nanoparticles with luminescence property (Zhang et al. 2019a ), And detection of harmful N-nitrosamines with the utilization of magnetic nanoparticles (Miralles et al. 2019 ) are worth mentioning.
Oil industry benefits from multiple types of nanomaterials
Nanomaterials can play a major role in the advancement of the oil industry. Almost every form of nanomaterial—discussed in previous sections—has been exhibited to have numerous applications in the oil industry. Nanomaterial can be effectively exploited in various processes of this industry, including oil exploration/production and recovering the oilfield. Nanofluids (synthesized from nanomaterials) optimize the oil production process. Nanocatalysts have applications in petrochemical processes along with operating an efficient oil purgation function. Several applications of this technology are mentioned below.
There are nanomembranes designed to provide a proper matrix for separating water and oil from gas. They eventually purify the gas and delete redundant components from wastewater (Saleh 2018 ). Metal workings such as machining and stamping industry require some types of lubricants and coolants, which are mostly oil products. There has been produced an oil-based cutting fluid made up of Al 2 O 3 nanoparticles to decrease the friction force between the object and snipping tool (Subhedar et al. 2021 ). Encapsulation of extracted essential oil from hyssop in a nano-complex improves the antioxidant and antifungal efficiency of the oil (Hadidi et al. 2021 ). The application of nano-silica in the procedure of oil cementing enhances the resistance of the cement (Goyal et al. 2021 ; Thakkar et al. 2020 ). In the process of oil recovery, there is a high energy loss that imposes damages to the injection system and lowers the heat level. To keep the rate of temperature in a higher range and decrease the energy loss, scientists have applied nano-thermal insulators that are more economical (Afra et al. 2021 ; Zhao et al. 2021 ; Zhou et al. 2020 ). Gas and oil products can be cleaned from H 2 S by applying nanomaterials (Agarwal and Sudharsan 2021 ). Utilizing starch nano coatings (Wang et al. 2021 ), Lignin and nano-silica (Gong et al. 2021 ), Lotus leaf coated with nano-SiO 2 (Yang et al. 2021 ), and nano zeolite membrane are new methods for the separation of oil and water due to their high hydrophobic property (Anis et al. 2021 ). Nanotechnology can be used to improve the quality of engine oil, which results in the better stability and lubricity power as well as a reduced rate of released carbon mono oxide (Tonk 2021 ; Saidi et al. 2021 ; Thirugnanam et al. 2021 ; Ardebili et al. 2020 ). Advanced nanoemulsions show high stability and benefits for the oil industry due to the larger surface and the ability to wet (Kumar et al. 2021 ). Encapsulation of essential oils in nanostructures indicates a better performance as a pesticide due to better maintenance of the oil (Campolo et al. 2020 ). Producing an oil-in-water emulsion by applying protein nanoparticles can protect unstable and active ingredients and benefit the medicine and food industry (Xu et al. 2020 ).
Combining diverse fields of science in a manner that they overcome each other’s deficiencies indicates promising results. Within the last decades, biotechnology has made a lot of progress. Merging nanotechnology with biotechnological methods enables scientists to design less time taking, more economical, and more efficient techniques. This Nano-biotechnological approach influences multiple therapeutic, agricultural, environmental, and industrial methods. For instance, the effectiveness of the emergent crisper/cas9 systems increases noticeably by applying the nano-scaled additives at the process.
In this review, we investigated the current advancements and limitations of biotechnology, along with the nano-based alternatives rendered by nanotechnology. It seems highly probable that biotechnology will accomplish even more improvements in the future, and its incorporation with nanotechnology gets humankind one step closer to a sustainable future. Besides, the nano-based techniques are less costly compared to the conventional ones. Thus, with nano-biotechnology promoting, a revolution in the economic situation of the world is not implausible.
Abass OK, Zhang K (2020) Nano-Fe mediated treatment of real hydraulic fracturing flowback and its practical implication on membrane fouling in tandem anaerobic-oxic membrane bioreactor. J Hazard Mater 395:122666
CAS PubMed Google Scholar
Abdelhamid HN, Badr G (2021) Nanobiotechnology as a platform for the diagnosis of COVID-19: a review. Nanotechnol Environ Eng 6(1):1–26
CAS Google Scholar
Adam H, Youssef A (2019) Economic impacts of nanotechnology industry: case study on Egypt. In: Proceedings of 10th International Conference on Digital Strategies for Organizational Success. https://doi.org/10.2139/ssrn.3314931
Adeel M, Bilal M, Rasheed T, Sharma A, Iqbal HMN (2018) Graphene and graphene oxide: functionalization and nano-bio-catalytic system for enzyme immobilization and biotechnological perspective. Int J Biol Macromol 120(Pt B):1430–1440
Afra M, Peyghambarzadeh SM, Shahbazi K, Tahmassebi N (2021) Thermo-economic optimization of steam injection operation in enhanced oil recovery (EOR) using nano-thermal insulation. Energy 226:120409
Agarwal M, Sudharsan J (2021) A comprehensive review on scavenging H 2 S compounds in oil and gas industry by using nanomaterials. Mater Today Proc. https://doi.org/10.1016/j.matpr.2020.11.693
Article PubMed PubMed Central Google Scholar
Ahire JJ, Dicks LMT (2014) 2,3-dihydroxybenzoic acid-containing nanofiber wound dressings inhibit biofilm formation by Pseudomonas aeruginosa . Antimicrob Agents Chemother 58(4):2098–2104
PubMed PubMed Central Google Scholar
Ahire JJ, Dicks LM (2016) Antimicrobial hyaluronic acid–cefoxitin sodium thin films produced by electrospraying. Curr Microbiol 73(2):236–241
Ahire JJ, Neveling DP (2018) Dicks LM (2018) Polyacrylonitrile (PAN) nanofibres spun with copper nanoparticles: an anti- Escherichia coli membrane for water treatment. Appl Microbiol Biotechnol 102(16):7171–7181
Ahire JJ, Hattingh M, Neveling DP, Dicks LM (2016) Copper-containing anti-biofilm nanofiber scaffolds as a wound dressing material. PLoS ONE 11(3):e0152755
Ahire JJ, Robertson DD, van Reenen AJ, Dicks LMT (2017a) Polyethylene oxide (PEO)-hyaluronic acid (HA) nanofibers with kanamycin inhibits the growth of Listeria monocytogenes. Biomed Pharmacother 86:143–148
Ahire JJ, Robertson DD, Van Reenen AJ, Dicks LM (2017b) Surfactin-loaded polyvinyl alcohol (PVA) nanofibers alters adhesion of Listeria monocytogenes to polystyrene. Mater Sci Eng C 77:27–33
Ahmadi MH, Ghazvini M, Alhuyi Nazari M, Ahmadi MA, Pourfayaz F, Lorenzini G, Ming T (2019) Renewable energy harvesting with the application of nanotechnology: a review. Int J Energy Res 43(4):1387–1410
Google Scholar
Al-Attabi R, Rodriguez-Andres J, Schütz JA, Bechelany M, Des Ligneris E, Chen X, Kong L, Morsi YS, Dumee LF (2019) Catalytic electrospun nano-composite membranes for virus capture and remediation. Sep Purif Technol 229:115806
Al-Gheethi AAS, Mohamed RMSR, Noman EA, Kassim AHM (eds) (2020) Prospects of fresh market wastes management in developing countries. Water science and technology library, vol 92. Springer, Cham
Ali M, Hoque M, Safdar Hossain S, Biswas M (2020) Nanoadsorbents for wastewater treatment: next generation biotechnological solution. Int J Environ Sci Technol 17:4095–4132
Ali SS, Al-Tohamy R, Koutra E, Moawad MS, Kornaros M, Mustafa AM, Mahmoud YA-G, Badr A, Osman ME, Elsamahy T (2021) Nanobiotechnological advancements in agriculture and food industry: applications, nanotoxicity, and future perspectives. Sci Total Environ 792:148359
AlNadhari S, Al-Enazi NM, Alshehrei F, Ameen F (2021) A review on biogenic synthesis of metal nanoparticles using marine algae and its applications. Environ Res 194:110672
Anis SF, Lalia BS, Lesimple A, Hashaikeh R, Hilal N (2021) Superhydrophilic and underwater superoleophobic nano zeolite membranes for efficient oil-in-water nanoemulsion separation. J Water Process Eng 40:101802
Ardebili SMS, Taghipoor A, Solmaz H, Mostafaei M (2020) The effect of nano-biochar on the performance and emissions of a diesel engine fueled with fusel oil-diesel fuel. Fuel 268:117356
Ashraf S, Siddiqa A, Shahida S, Qaisar S (2019) Titanium-based nanocomposite materials for arsenic removal from water: a review. Heliyon 5(5):e01577
Azarakhsh F, Divsalar A, Saboury AA, Eidi A (2021) Simultaneous Delivery of Oxali-palladium and Iron Nanoparticles by β-Casein. Journal of Molecular Liquids:115999
Azeez NA, Dash SS, Gummadi SN, Deepa VS (2020) Nano-remediation of toxic heavy metal contamination: Hexavalent chromium [Cr (VI)]. Chemosphere:129204
Banerjee S, Dubey S, Gautam RK, Chattopadhyaya M, Sharma YC (2019) Adsorption characteristics of alumina nanoparticles for the removal of hazardous dye, Orange G from aqueous solutions. Arab J Chem 12(8):5339–5354
Barani M, Bilal M, Sabir F, Rahdar A, Kyzas GZ (2021) Nanotechnology in ovarian cancer: diagnosis and treatment. Life Sci 266:118914
Bashir F, Irfan M, Ahmad T, Iqbal J, Butt MT, Sadef Y, Umbreen M, Shaikh IA, Moniruzzaman M (2021) Efficient utilization of low cost agro materials for incorporation of copper nanoparticles to scrutinize their antibacterial properties in drinking water. Environ Technol Innov 21:101228
Bayda S, Adeel M, Tuccinardi T, Cordani M, Rizzolio F (2020) The history of nanoscience and nanotechnology: from chemical–physical applications to nanomedicine. Molecules 25(1):112
Benrabah L, Kemel K, Twarog C, Huang N, Solgadi A, Laugel C, Faivre V (2020) Lipid-based Janus nanoparticles for pharmaceutical and cosmetic applications: kinetics and mechanisms of destabilization with time and temperature. Colloids Surfaces B 195:111242
Bensaida K, Eljamal R, Sughihara Y, Eljamal O (2021) The impact of iron bimetallic nanoparticles on bulk microbial growth in wastewater. J Water Process Eng 40:101825
Beryani A, Pardakhti A, Ardestani M, Zahed MA (2017) Benzene and MTBE removal by Fenton’s process using stabilized Nano Zero-Valent Iron particles. J Appl Res Water Wastewater 4(2):343–348. https://doi.org/10.22126/arww.2017.788
Article Google Scholar
Bhuyan D, Greene GW, Das RK (2019) Prospects and application of nanobiotechnology in food preservation: molecular perspectives. Crit Rev Biotechnol 39(6):759–778
PubMed Google Scholar
Biesiada M, Pachulska-Wieczorek K, Adamiak RW, Purzycka KJ (2016) RNAComposer and RNA 3D structure prediction for nanotechnology. Methods 103:120–127
Borrás MC, Sluyter R, Barker PJ, Konstantinov K, Bakand S (2020) Y2O3 decorated TiO2 nanoparticles: enhanced UV attenuation and suppressed photocatalytic activity with promise for cosmetic and sunscreen applications. J Photochem Photobiol B Biol 207:111883
Campolo O, Giunti G, Laigle M, Michel T, Palmeri V (2020) Essential oil-based nano-emulsions: effect of different surfactants, sonication and plant species on physicochemical characteristics. Ind Crops Prod 157:112935
Cardoso VF, Francesko A, Ribeiro C, Bañobre-López M, Martins P, Lanceros-Mendez S (2018) Advances in magnetic nanoparticles for biomedical applications. Adv Healthcare Mater 7(5):1700845
Chai JM, Amelia TSM, Mouriya GK, Bhubalan K, Amirul A-AA, Vigneswari S, Ramakrishna S (2021) Surface-Modified highly biocompatible bacterial-poly (3-hydroxybutyrate-co-4-hydroxybutyrate): a review on the promising next-generation biomaterial. Polymers 13(1):51
Chen H, Gu L, Liao B, Zhou X, Cheng L, Ren B (2020) Advances of anti-caries nanomaterials. Molecules 25(21):5047
CAS PubMed Central Google Scholar
Chen L, Ai J, Cai H, Chen X, Liu Z, Li Z, Dai F (2021a) Antibacterial gauze based on the synergistic antibacterial mechanism of antimicrobial peptides and silver nanoparticles. J Polym Res 28(2):1–9
Chen Z-Y, Gupta A, Chattopadhyay S (2021b) Detection of mercury in spiked cosmetics by surface enhanced Raman spectroscopy using silver shelled iron oxide nanoparticles. Sens Actuators B Chem 337:129788
Chhipa H (2019) Applications of nanotechnology in agriculture. Methods Microbiol 46:115–142
Chiari-Andréo BG, Almeida-Cincotto MGJd, Oshiro JA, Taniguchi CYY, Chiavacci LA, Isaac VLB (2019) Chapter 5—Nanoparticles for cosmetic use and its application. In: Grumezescu AM (ed) Nanoparticles in pharmacotherapy. William Andrew Publishing, pp 113–146
Chugh G, Siddique KH, Solaiman ZM (2021) Nanobiotechnology for agriculture: smart technology for combating nutrient deficiencies with nanotoxicity challenges. Sustainability 13(4):1781
Costa JSD, Hoskisson PA, Paterlini P, Romero CM, Alvarez A (2020) Whole genome sequence of the multi-resistant plant growth-promoting bacteria Streptomyces sp. Z38 with potential application in agroindustry and bio-nanotechnology. Genomics 112(6):4684–4689
D’Acunto M, Cioni P, Gabellieri E, Presciuttini G (2021) Exploiting gold nanoparticles for diagnosis and cancer treatments. Nanotechnology 32(19):192001
de Azevedo Stavale A, Fonseca GO, Duarte PS, Macedo LC, Percebom AM (2019) Nanoparticles of surfactant and block copolymers with high uptake of oily ingredients for cosmetic formulations. Colloids Surf A Physicochem Eng Asp 581:123779
De Luca I, Pedram P, Moeini A, Cerruti P, Peluso G, Di Salle A, Germann N (2021) Nanotechnology development for formulating essential oils in wound dressing materials to promote the wound-healing process: a review. Appl Sci 11(4):1713
Debnath M, Chauhan N, Sharma P, Tomar I (2021) Potential of nano biosurfactants as an ecofriendly green technology for bioremediation. Handbook of nanomaterials for wastewater treatment. Elsevier, pp 1039–1055
Deng H, Huang W, Zhang Z (2019) Nanotechnology based CRISPR/Cas9 system delivery for genome editing: progress and prospect. Nano Res 12(10):2437–2450
Desai AV, Sharma S, Ghosh SK (2019) Metal-organic frameworks for recognition and sequestration of toxic anionic pollutants. Metal-organic frameworks (MOFs) for environmental applications. Elsevier, pp 95–140
Ding S-s, He L, Bian X-w, Tian G (2020) Metal-organic frameworks-based nanozymes for combined cancer therapy. Nano Today 35:100920
Doroudian M, MacLoughlin R, Poynton F, Prina-Mello A, Donnelly SC (2019) Nanotechnology based therapeutics for lung disease. Thorax 74(10):965–976
Doroudian M, O’Neill A, Mac Loughlin R, Prina-Mello A, Volkov Y, Donnelly SC (2021) Nanotechnology in pulmonary medicine. Curr Opin Pharmacol 56:85–92
Du T, Zhang J, Li C, Song T, Li P, Liu J, Du X, Wang S (2020) Gold/silver hybrid nanoparticles with enduring inhibition of coronavirus multiplication through multisite mechanisms. Bioconjug Chem 31(11):2553–2563
Ebrahimbabaie P, Meeinkuirt W, Pichtel J (2020) Phytoremediation of engineered nanoparticles using aquatic plants: mechanisms and practical feasibility. J Environ Sci 93:151–163
Evelyn Roopngam P (2019) Liposome and polymer-based nanomaterials for vaccine applications. Nanomed J 6(1):1–10
Farrera C, Torres Andón F, Feliu N (2017) Carbon nanotubes as optical sensors in biomedicine. ACS Nano 11(11):10637–10643
Fidoski J, Benedetti A, Kirkov A, Iliev A, Stamatoski A, Baftijari D (2020) Nano-emulsion complex (propolis and vitamin C) promotes wound healing in the oral mucosa. Oral Maxillofac Patho J 11(1):1–5
Fouad GI (2021) A proposed insight into the anti-viral potential of metallic nanoparticles against novel coronavirus disease-19 (COVID-19). Bull Natl Res Cent 45(1):1–22
Furtado D, Björnmalm M, Ayton S, Bush AI, Kempe K, Caruso F (2018) Overcoming the blood–brain barrier: the role of nanomaterials in treating neurological diseases. Adv Mater 30(46):1801362
Gangadoo S, Nguyen H, Rajapaksha P, Zreiqat H, Latham K, Cozzolino D, Truong VK (2021) Inorganic nanoparticles as food additives and their influence on the human gut microbiota. Environ Sci Nano 8:1500–1518
Ghebretatios M, Schaly S, Prakash S (2021) Nanoparticles in the food industry and their impact on human gut microbiome and diseases. Int J Mol Sci 22(4):1942
CAS PubMed PubMed Central Google Scholar
Gökçe D (2021) Influences of nanoparticles on aquatic organisms: current situation of nanoparticles effects in aquatic ecosystems. Sust Eng Innov 3(1):54–60
Gong X, Meng Y, Zhu J, Wang X, Lu J, Cheng Y, Tao Y, Wang H (2021) Construct a stable super-hydrophobic surface through acetonitrile extracted lignin and nano-silica and its application in oil-water separation. Ind Crops Prod 166:113471
González-Ballesteros N, Prado-López S, Rodríguez-González J, Lastra M, Rodríguez-Argüelles M (2017) Green synthesis of gold nanoparticles using brown algae Cystoseira baccata : Its activity in colon cancer cells. Colloids Surf B 153:190–198
Goracci M, Pignochino Y, Marchiò S (2020) Phage display-based nanotechnology applications in cancer immunotherapy. Molecules 25(4):843
Goyal S, Joshi P, Singh R (2021) Applications and role of Nano-Silica particles on altering the properties and their usage for oil well cementing. Mater Today Proc 46:10681–10686
Hadidi M, Motamedzadegan A, Jelyani AZ, Khashadeh S (2021) Nanoencapsulation of hyssop essential oil in chitosan-pea protein isolate nano-complex. LWT 144:111254
Hager S, Fittler FJ, Wagner E, Bros M (2020) Nucleic acid-based approaches for tumor therapy. Cells 9(9):2061
Hajiabadi SH, Aghaei H, Kalateh-Aghamohammadi M, Shorgasthi M (2020) An overview on the significance of carbon-based nanomaterials in upstream oil and gas industry. J Pet Sci Eng 186:106783
He Y, Lin H, Luo M, Liu J, Dong Y, Li B (2020) Highly efficient remediation of groundwater co-contaminated with Cr (VI) and nitrate by using nano-Fe/Pd bimetal-loaded zeolite: process product and interaction mechanism. Environ Pollut 263:114479
Hosseini SF, Ramezanzade L, McClements DJ (2021) Recent advances in nanoencapsulation of hydrophobic marine bioactives: bioavailability, safety, and sensory attributes of nano-fortified functional foods. Trends Food Sci Technol 109:322–339
Hou S, Wu B, Peng D, Wang Z, Wang Y, Xu H (2019) Remediation performance and mechanism of hexavalent chromium in alkaline soil using multi-layer loaded nano-zero-valent iron. Environ Pollut 252:553–561
Islam MA, Rice J, Reesor E, Zope H, Tao W, Lim M, Ding J, Chen Y, Aduluso D, Zetter BR (2021) Adjuvant-pulsed mRNA vaccine nanoparticle for immunoprophylactic and therapeutic tumor suppression in mice. Biomaterials 266:120431
Iwaniec DM, Cook EM, Davidson MJ, Berbés-Blázquez M, Georgescu M, Krayenhoff ES, Middel A, Sampson DA, Grimm NB (2020) The co-production of sustainable future scenarios. Landsc Urban Plan 197:103744
Jafari SM, Arpagaus C, Cerqueira MA, Samborska K (2021) Nano spray drying of food ingredients; materials, processing and applications. Trends Food Sci Technol 109:632–646
Jang W, Kwon H, Park Y, Lee H (2018) Predicting the degree of interdisciplinarity in academic fields: the case of nanotechnology. Scientometrics 116(1):231–254
Jeevanandam J, Pal K, Danquah MK (2019) Virus-like nanoparticles as a novel delivery tool in gene therapy. Biochimie 157:38–47
Jeong YJ, Kim DS, Kim JY, Oyunbaatar NE, Shanmugasundaram A, Kim ES, Lee DW (2021) On-stage bioreactor platform integrated with nano-patterned and gold-coated PDMS diaphragm for live cell stimulation and imaging. Mater Sci Eng C-Mater Biol Appl 118:9. https://doi.org/10.1016/j.msec.2020.111355
Article CAS Google Scholar
Jiang Y, Khan A, Huang H, Tian Y, Yu X, Xu Q, Mou L, Lv J, Zhang P, Liu P, Deng L, Li X (2019) Using nano-attapulgite clay compounded hydrophilic urethane foams (AT/HUFs) as biofilm support enhances oil-refinery wastewater treatment in a biofilm membrane bioreactor. Sci Total Environ 646:606–617
Jogee P, Agarkar G, Rai M (2021) Starch-based films loaded with nano-antimicrobials for food packaging. Biopolymer-based nano films. Elsevier, pp 99–114
Jun B-M, Al-Hamadani YA, Son A, Park CM, Jang M, Jang A, Kim NC, Yoon Y (2020) Applications of metal-organic framework based membranes in water purification: a review. Sep Purif Technol 247:116947
Kailasa SK, Mehta VN, Koduru JR, Basu H, Singhal RK, Murthy Z, Park T-J (2021) An overview of molecular biology and nanotechnology based analytical methods for the detection of SARS-CoV-2: promising biotools for the rapid diagnosis of COVID-19. Analyst 146(5):1489–1513
Karimzadeh Z, Javanbakht S, Namazi H (2019) Carboxymethylcellulose/MOF-5/Graphene oxide bio-nanocomposite as antibacterial drug nanocarrier agent. BioImpacts 9(1):5
Ken DS, Sinha A (2020) Recent developments in surface modification of nano zero-valent iron (nZVI): remediation, toxicity and environmental impacts. Environ Nanotechnol Monit Manag 24:100344
Khezri K, Saeedi M, Dizaj SM (2018) Application of nanoparticles in percutaneous delivery of active ingredients in cosmetic preparations. Biomed Pharmacother 106:1499–1505
Kim S, Kim K, Jun G, Hwang W (2020) Wood-nanotechnology-based membrane for the efficient purification of oil-in-water emulsions. ACS Nano 14(12):17233–17240
Kinik FP, Kampouri S, Ebrahim FM, Valizadeh B, Stylianou KC (2020) Porous metal-organic frameworks for advanced applications. Elsevier
Kinloch IA, Suhr J, Lou J, Young RJ, Ajayan PM (2018) Composites with carbon nanotubes and graphene: an outlook. Science 362(6414):547–553
Korde JM, Kandasubramanian B (2020) Microbiologically extracted poly (hydroxyalkanoates) and its amalgams as therapeutic nano-carriers in anti-tumor therapies. Mater Sci Eng C 111:110799
Kumar S, Kashyap PL, Mahapatra S, Jasrotia P, Singh GP (2020) New and emerging technologies for detecting Magnaporthe oryzae causing blast disease in crop plants. Crop Prot 143:105473
Kumar N, Verma A, Mandal A (2021) Formation, characteristics and oil industry applications of nanoemulsions: a review. J Pet Sci Eng 206:109042
Kutara BMRNS, Sutharb H, Mohitec KC, Devid R (2016) Bioinformatics, biotechnology and nanotechnology tools for environment engineering: way to sustainable development by wastewater treatment
Landowski LM, Niego B, Sutherland BA, Hagemeyer CE, Howells DW (2020) Applications of nanotechnology in the diagnosis and therapy of stroke. Semin Thromb Hemost 46(5):592–605. https://doi.org/10.1055/s-0039-3399568
Article CAS PubMed Google Scholar
Le TT, Yoon H, Son M-H, Kang Y-G, Chang Y-S (2019) Treatability of hexabromocyclododecane using Pd/Fe nanoparticles in the soil-plant system: effects of humic acids. Sci Total Environ 689:444–450
Lew TTS, Koman VB, Gordiichuk P, Park M, Strano MS (2020) The emergence of plant nanobionics and living plants as technology. Adv Mater Technol 5(3):1900657
Li T, Fang F, Yang Y, Shen W, Bao W, Zhang T, Ai F, Ding X, Xin H, Wang X (2019) Surface nano-engineered wheat straw for portable and adjustable water purification. Sci Total Environ 655:1028–1036
Li R, Ng TS, Wang SJ, Prytyskach M, Rodell CB, Mikula H, Kohler RH, Garlin MA, Lauffenburger DA, Parangi S (2021) Therapeutically reprogrammed nutrient signalling enhances nanoparticulate albumin bound drug uptake and efficacy in KRAS-mutant cancer. Nat Nanotechnol. https://doi.org/10.1038/s41565-021-00897-1
Lian M, Wang L, Feng Q, Niu L, Zhao Z, Wang P, Song C, Li X, Zhang Z (2021) Thiol-functionalized nano-silica for in-situ remediation of Pb, Cd, Cu contaminated soils and improving soil environment. Environ Pollut 280:116879
Lim M, Badruddoza AZM, Firdous J, Azad M, Mannan A, Al-Hilal TA, Cho C-S, Islam MA (2020) Engineered nanodelivery systems to improve DNA vaccine technologies. Pharmaceutics 12(1):30
Lin Y-X, Wang Y, Blake S, Yu M, Mei L, Wang H, Shi J (2020) RNA nanotechnology-mediated cancer immunotherapy. Theranostics 10(1):281–299. https://doi.org/10.7150/thno.35568
Article CAS PubMed PubMed Central Google Scholar
Lincy A, Jegathambal P, Mkandawire M, MacQuarrie S (2020) Nano bioremediation of textile dye effluent using magnetite nanoparticles encapsulated alginate beads. J Environ Treat Tech 8(3):936–946
Liu B, Hu F, Zhang JF, Wang CL, Li LL (2019) A biomimetic coordination nanoplatform for controlled encapsulation and delivery of drug-gene combinations. Angew Chem-Int Edit 58(26):8804–8808
Lohrasebi A, Koslowski T (2019) Modeling water purification by an aquaporin-inspired graphene-based nano-channel. J Mol Model 25(9):280
Lombardo D, Kiselev MA, Caccamo MT (2019) Smart nanoparticles for drug delivery application: development of versatile nanocarrier platforms in biotechnology and nanomedicine. J Nanomater. https://doi.org/10.1155/2019/3702518
Luo Y, Wang Q, Zhang Y (2020) Biopolymer-based nanotechnology approaches to deliver bioactive compounds for food applications: a perspective on the past, present, and future. J Agric Food Chem 68(46):12993–13000
Luo Z, Zhu J, Yu L, Yin K (2021) Heavy metal remediation by nano zero-valent iron in the presence of microplastics in groundwater: inhibition and induced promotion on aging effects. Environ Pollut 287:117628
Lyu P, Javidi-Parsijani P, Atala A, Lu B (2019) Delivering Cas9/sgRNA ribonucleoprotein (RNP) by lentiviral capsid-based bionanoparticles for efficient ‘hit-and-run’genome editing. Nucleic Acids Res 47(17):e99–e99
Ma J, Dai R, Chen M, Khan SJ, Wang Z (2018) Applications of membrane bioreactors for water reclamation: micropollutant removal, mechanisms and perspectives. Biores Technol 269:532–543
Mahmoud ME, Allam EA, El-Sharkawy RM, Soliman MA, Saad EA, El-Khatib AM (2020) Nano-manganese oxide-functionalized-oleyl amine as a simple and low cost nanosorbent for remediation of ZnII/CoII and their radioactive nuclides 65Zn and 60Co from water. Appl Radiat Isot 159:108989
Maine E, Thomas V, Bliemel M, Murira A, Utterback J (2014) The emergence of the nanobiotechnology industry. Nat Nanotechnol 9(1):2–5
Majhi RK, Mohanty S, Khan MI, Mishra A, Brauner A (2021) Ag@ ZnO nanoparticles induce antimicrobial peptides and promote migration and antibacterial activity of keratinocytes. ACS Infect Dis. https://doi.org/10.1021/acsinfecdis.0c00903
Malik SN, Khan SM, Ghosh PC, Vaidya AN, Kanade G, Mudliar SN (2019) Treatment of pharmaceutical industrial wastewater by nano-catalyzed ozonation in a semi-batch reactor for improved biodegradability. Sci Total Environ 678:114–122
Manikanika KJ, Jaswal S (2021) Role of nanotechnology in the world of cosmetology: a review. Mater Today Proc. https://doi.org/10.1016/j.matpr.2020.12.638
Mantatov V, Mantatova L, Nasibulina A, Tutubalin V (2019) The conception of sustainable development in the light of converging technologies. Eur Asian J Bio Sci 13(2):2159–2165
Marchiol L (2018) Nanotechnology in agriculture: new opportunities and perspectives. New Visions Plant Sci 9(4):161
Marcos-Contreras OA, Greineder CF, Kiseleva RY, Parhiz H, Walsh LR, Zuluaga-Ramirez V, Myerson JW, Hood ED, Villa CH, Tombacz I, Pardi N, Seliga A, Mui BL, Tam YK, Glassman PM, Shuvaev VV, Nong J, Brenner JS, Khoshnejad M, Madden T, Weissmann D, Persidsky Y, Muzykantov VR (2020) Selective targeting of nanomedicine to inflamed cerebral vasculature to enhance the blood–brain barrier. Proc Natl Acad Sci 117(7):3405–3414
Meikle TG, Dharmadana D, Hoffmann SV, Jones NC, Drummond CJ, Conn CE (2021) Analysis of the structure, loading and activity of six antimicrobial peptides encapsulated in cubic phase lipid nanoparticles. J Colloid Interface Sci 587:90–100
Milinčić DD, Popović DA, Lević SM, Kostić A, Tešić ŽL, Nedović VA, Pešić MB (2019) Application of polyphenol-loaded nanoparticles in food industry. Nanomaterials. https://doi.org/10.3390/nano9111629
Miralles P, van Gemert I, Chisvert A, Salvador A (2019) Stir bar sorptive-dispersive microextraction mediated by magnetic nanoparticles-metal organic framework composite: determination of N-nitrosamines in cosmetic products. J Chromatogr A 1604:460465
Mishra R, Manral A (2021) Graphene functionalized starch biopolymer nanocomposites: fabrication, characterization, and applications. Graphene based biopolymer nanocomposites. Springer, pp 173–189
Mishra A, Kumar J, Melo JS, Sandaka BP (2021) Progressive development in biosensors for detection of dichlorvos pesticide: a review. J Environ Chem Eng 9(2):105067
Mofijur M, Siddiki SYA, Ahmed MB, Djavanroodi F, Fattah IR, Ong HC, Chowdhury M, Mahlia T (2020) Effect of nanocatalysts on the transesterification reaction of first, second and third generation biodiesel sources-A mini-review. Chemosphere 270:128642
Mohid SA, Bhunia A (2021) Combining antimicrobial peptides with nanotechnology: an emerging field in theranostics. Curr Protein Pept Sci 21(4):413–428
Murshid S, Gopinath KP, Prakash DG (2021) Current nanotechnology based solutions for sustainable wastewater treatment. Curr Anal Chem 17(2):166–184
Nasrollahzadeh M, Sajjadi M, Iravani S, Varma RS (2020) Green-synthesized nanocatalysts and nanomaterials for water treatment: current challenges and future perspectives. J Hazard Mater 401:123401
Nawaz MZ, Attique SA, Alshammari FA, Alhamdi HW, Alghamdi HA, Yan W (2021) A nanoinformatics approach to evaluate the pharmacological properties of nanoparticles for the treatment of Alzheimer’s disease. Comb Chem High Throughput Screening. https://doi.org/10.2174/1386207324666210217145733
Ndlovu N, Mayaya T, Muitire C, Munyengwa N (2020) Nanotechnology applications in crop production and food systems. Int J Plant Breeding Crop Sci 7(1):624–634
Nehra M, Dilbaghi N, Marrazza G, Kaushik A, Sonne C, Kim K-H, Kumar S (2021) Emerging nanobiotechnology in agriculture for the management of pesticide residues. J Hazard Mater 401:123369
Nguyen VT, Vu QH, Pham TN, Trinh KS (2021) Antibacterial filtration using polyethylene terephthalate filters coated with copper nanoparticles. J Nanomater. https://doi.org/10.1155/2021/6628362
Nizami A-S, Rehan M (2018) Towards nanotechnology-based biofuel industry. Biofuel Res J 5(2):798
Oliveira AG, de Lara AJ, Montanha MC, Ogawa CYL, de Souza Freitas TKF, Moraes JCG, Sato F, Lima SM, da Cunha Andrade LH, Hechenleitner AAW (2021) Wastewater treatment using Mg-doped ZnO nano-semiconductors: a study of their potential use in environmental remediation. J PhotochemPhotobiol A Chem 407:113078
Ozogul F, Elabed N, Ceylan Z, Ocak E, Ozogul Y (2021) Nano-technological approaches for plant and marine-based polysaccharides for nano-encapsulations and their applications in food industry. Elsevier, pp 187–236
Paiva-Santos AC, Herdade AM, Guerra C, Peixoto D, Pereira-Silva M, Zeinali M, Mascarenhas-Melo F, Paranhos A, Veiga F (2021) Plant-mediated green synthesis of metal-based nanoparticles for dermopharmaceutical and cosmetic applications. Int J Pharm 597:120311
Patel RM, Dahane TM, Godbole S, Kambala SS, Mangal K (2020) Applications of nanotechnology in prosthodontics. J Evol Med Dent Sci 9(47):3566–3572
Patra Shahi M, Kumari P, Mahobiya D, Kumar Shahi S (2021) Chapter 4—nano-bioremediation of environmental contaminants: applications, challenges, and future prospects. In: Kumar V, Saxena G, Shah MP (eds) Bioremediation for environmental sustainability. Elsevier, pp 83–98
Paunovska K, Loughrey D, Sago CD, Langer R, Dahlman JE (2019) Using large datasets to understand nanotechnology. Adv Mater 31(43):1902798
Peer D, Karp JM, Hong S, Farokhzad OC, Margalit R, Langer R (2020) Nanocarriers as an emerging platform for cancer therapy. Nano-enabled medical applications. Jenny Stanford Publishing, pp 61–91
Rabiee N, Bagherzadeh M, Ghasemi A, Zare H, Ahmadi S, Fatahi Y, Dinarvand R, Rabiee M, Ramakrishna S, Shokouhimehr M (2020) Point-of-use rapid detection of sars-cov-2: nanotechnology-enabled solutions for the COVID-19 pandemic. Int J Mol Sci 21(14):5126
Raghav S, Yadav PK, Kumar D (2020) Nanotechnology for a sustainable future. Handbook of nanomaterials for manufacturing applications. Elsevier, pp 465–492
Razavi R, Kenari RE, Farmani J, Jahanshahi M (2020) Fabrication of zein/alginate delivery system for nanofood model based on pumpkin. Int J Biol Macromol 165(Pt B):3123–3134
Reshmy R, Philip E, Madhavan A, Sindhu R, Pugazhendhi A, Binod P, Sirohi R, Awasthi MK, Tarafdar A, Pandey A (2021) Advanced biomaterials for sustainable applications in the food industry: updates and challenges. Environ Pollut 283:117071
Rezaei M, Shokuhfar A, Shahcheraghi N (2021) Preparation and photocatalytic performance of flower-shaped Zno/GO/Fe 3 O 4 on degradation of rhodamine B. Defect and diffusion forum. Trans Tech Publications, pp 161–172
Roco MC, Bainbridge WS (2013) Converging technologies for improving human performance: nanotechnology, biotechnology, information technology and cognitive science. Springer Science Business Media
Rottenberg S, Disler C, Perego P (2021) The rediscovery of platinum-based cancer therapy. Nat Rev Cancer 21(1):37–50
Rovera C, Ghaani M, Farris S (2020) Nano-inspired oxygen barrier coatings for food packaging applications: an overview. Trends Food Sci Technol 97:210–220
Ruan X, Liu D, Niu X, Wang Y, Simpson CD, Cheng N, Du D, Lin Y (2019) 2D Graphene oxide/Fe-MOF nanozyme nest with superior peroxidase-like activity and its application for detection of woodsmoke exposure biomarker. Anal Chem 91(21):13847–13854
Saari NHM, Chua LS (2020) Nano-based products in beverage industry. Nanoengineering in the beverage industry. Elsevier, pp 405–436
Sahani S, Sharma YC (2020) Advancements in applications of nanotechnology in global food industry. Food Chem 342:128318
Sahu J, Karri RR, Zabed HM, Shams S, Qi X (2021) Current perspectives and future prospects of nano-biotechnology in wastewater treatment. Sep Purif Rev 50(2):139–158
Saidi MZ, El Moujahid C, Pasc A, Canilho N, Delgado-Sanchez C, Celzard A, Fierro V, Kouitat-Njiwa R, Chafik T (2021) Enhanced tribological properties of wind turbine engine oil formulated with flower-shaped MoS2 nano-additives. Colloids Surfaces A 620:126509
Sajjadi M, Ahmadpoor F, Nasrollahzadeh M, Ghafuri H (2021) Lignin-derived (nano) materials for environmental pollution remediation: current challenges and future perspectives. Int J Biol Macromol 178:394–423
Sakulwech S, Lourith N, Ruktanonchai U, Kanlayavattanakul M (2018) Preparation and characterization of nanoparticles from quaternized cyclodextrin-grafted chitosan associated with hyaluronic acid for cosmetics. Asian J Pharm Sci 13(5):498–504
Saleh TA (2018) Nanotechnology in oil and gas industries. Springer, Cham
Saleh TA (2020) Nanomaterials: classification, properties, and environmental toxicities. Environ Technol Innov 20:101067
Samrot AV, Sahithya CS, Selvarani J, Purayil SK, Ponnaiah P (2020) A review on synthesis, characterization and potential biological applications of superparamagnetic iron oxide nanoparticles. Curr Res Green Sust Chem 4:100042
Sánchez-Rubio F, Soria-Meneses PJ, Jurado-Campos A, Bartolome-Garcia J, Gomez-Rubio V, Soler AJ, Arroyo-Jimenez M, Santander-Ortega M, Plaza-Oliver M, Lozano M (2020) Nanotechnology in reproduction: vitamin E nanoemulsions for reducing oxidative stress in sperm cells. Free Radical Biol Med 160:47–56
Santos AC, Morais F, Simões A, Pereira I, Sequeira JAD, Pereira-Silva M, Veiga F, Ribeiro A (2019) Nanotechnology for the development of new cosmetic formulations. Expert Opin Drug Deliv 16(4):313–330
Séby F (2021) Metal and metal oxide nanoparticles in cosmetics and skin care products. Elsevier
Selivanovitch E, Douglas T (2019) Virus capsid assembly across different length scales inspire the development of virus-based biomaterials. Curr Opin Virol 36:38–46
Sharma A, Singh G, Arya SK (2021) Biofuel cell nanodevices. Int J Hydrogen Energy 46(4):3270–3288
Singh R, Misra V, Mudiam MKR, Chauhan LKS, Singh RP (2012) Degradation of γ-HCH spiked soil using stabilized Pd/Fe0 bimetallic nanoparticles: pathways, kinetics and effect of reaction conditions. J Hazard Mater 237–238:355–364
Subhedar DG, Patel YS, Ramani BM, Patange GS (2021) An experimental investigation on the effect of Al 2 O 3 /cutting oil based nano coolant for minimum quantity lubrication drilling of SS 304. Cleaner Eng Technol 3:100104
Ta Q, Ting J, Harwood S, Browning N, Simm A, Ross K, Olier I, Al-Kassas R (2021) Chitosan nanoparticles for enhancing drugs and cosmetic components penetration through the skin. Eur J Pharm Sci 160:105765
Tan H, Wang C, Li H, Peng D, Zeng C, Xu H (2020) Remediation of hexavalent chromium contaminated soil by nano-FeS coated humic acid complex in combination with Cr-resistant microflora. Chemosphere 242:125251
Tang C-H (2021) Assembly of food proteins for nano-encapsulation and delivery of nutraceuticals (a mini-review). Food Hydrocoll 117:106710
Tavakoli N, Divsalar A, Haertlé T, Sawyer L, Saboury AA, Muronetz V (2021) Milk protein-based nanodelivery systems for the cancer treatment. J Nanost Chem 11:483–500
Thakkar A, Raval A, Chandra S, Shah M, Sircar A (2020) A comprehensive review of the application of nano-silica in oil well cementing. Petroleum 6(2):123–129
Thangadurai D, Sangeetha J, Prasad R (2020) Nanotechnology for food, agriculture, and environment. Springer International Publishing
Thirugnanam C, Dharmaprabhakaran T, Kalaimurugan K, Somasundaram D, Kumar AV, Ali MMJ (2021) Emission analysis of Palmyrah seed oil bio-diesel with NiO nano-particles on CI engine. Mater Today Proc. https://doi.org/10.1016/j.matpr.2021.01.87
Thomas S, Kumar Mishra P, Talegaonkar S (2015) Ceramic nanoparticles: fabrication methods and applications in drug delivery. Curr Pharm Des 21(42):6165–6188
Tiwari K, Singh R, Negi P, Dani R, Rawat A (2021) Application of nanomaterials in food packaging industry: a review. Mater Today Proc. https://doi.org/10.1016/j.matpr.2021.01.385
Tonk R (2021) The science and technology of using nano-materials in engine oil as a lubricant additives. Mater Today Proc 37:3475–3479
Usman M, Farooq M, Wakeel A, Nawaz A, Cheema SA, Ur Rehman H, Ashraf I, Sanaullah M (2020) Nanotechnology in agriculture: current status, challenges and future opportunities. Sci Total Environ 721:137778
Valencia PM, Farokhzad OC, Karnik R, Langer R (2020) Microfluidic technologies for accelerating the clinical translation of nanoparticles. Nano-enabled medical applications. Jenny Stanford Publishing, pp 93–112
Vázquez-Núñez E, Molina-Guerrero CE, Peña-Castro JM, Fernández-Luqueño F, de la Rosa-Álvarez M (2020) Use of nanotechnology for the bioremediation of contaminants: a review. Processes 8(7):826
Visentin C, da Silva Trentin AW, Braun AB, Thomé A (2020) Nano scale zero valent iron production methods applied to contaminated sites remediation: an overview of production and environmental aspects. J Hazard Mater 410:124614
Wang D, Chen Y (2016) Critical review of the influences of nanoparticles on biological wastewater treatment and sludge digestion. Crit Rev Biotechnol 36(5):816–828
Wang Y, Zhang G (2020) The preparation of modified nano-starch and its application in food industry. Food Res Int 140:110009
Wang S, Chen Y, Wang S, Li P, Mirkin CA, Farha OK (2019) DNA-functionalized metal–organic framework nanoparticles for intracellular delivery of proteins. J Am Chem Soc 141(6):2215–2219
Wang Y, Chen Y, Hu Y, Fang X (2020) Site-specific covalent labeling of large RNAs with nanoparticles empowered by expanded genetic alphabet transcription. Proc Natl Acad Sci 117(37):22823–22832
Wang F, Chang R, Ma R, Tian Y (2021) Eco-friendly and superhydrophobic nano-starch based coatings for self-cleaning application and oil-water separation. Carbohydr Polym 271:118410
Weijie M, Chongnv W, Xuming P, Weixin J, Yuhang W, Benhui S (2020) TiO2 nanoparticles and multi-walled carbon nanotubes monitoring and bioremediation potential using ciliates Pseudocohnilembus persalinus . Ecotoxicol Environ Saf 187:109825
Xu B, Liu C, Sun H, Wang X, Huang F (2020) Oil-in-water Pickering emulsions using a protein nano-ring as high-grade emulsifiers. Colloids Surfaces B 187:110646
Yadwade R, Gharpure S, Ankamwar B (2021) Nanotechnology in cosmetics pros and cons. Nano Express 2(2):022003
Yang Y, Li B, Peng J, Lei Z, Zhu E, Zhang X, Feng X, Mi Y (2021) The demulsification of crude oil emulsion driven by a natural lotus leaf grafted with nano-SiO2. J Environ Chem Eng 9(4):105586
Yao L, Ouyang L, Lv J, Dai P, Zhu L (2021) Rapid and sensitive SERS detection of food contaminants by using nano-Ag aggregates with controllable hydrophobicity. Microchem J 166:106221
Yue T, Liu J, Yang Y, Chen S (2021) Feasibility of cleaning As-and U-containing soil of a gold mine by using biologically nano-sized FeS: implications for soil remediation technology. Environ Technol Innov. https://doi.org/10.1016/j.eti.2021.101775
Zahed MA, Revayati M, Shahcheraghi N, Maghsoudi F, Tabari Y (2021a) Modeling and optimization of biodiesel synthesis using TiO2–ZnO nanocatalyst and characteristics of biodiesel made from waste sunflower oil. Curr Res Green Sustain Chem 4:100223
Zahed MA, Movahed E, Khodayari A, Zanganeh S, Badamaki M (2021b) Biotechnology for carbon capture and fixation: critical review and future directions. J Environ Manag 293:112830
Zhang S, Yao T, Wang S, Feng R, Chen L, Zhu V, Hu G, Zhang H, Yang G (2019a) Upconversion luminescence nanoparticles-based immunochromatographic assay for quantitative detection of triamcinolone acetonide in cosmetics. Spectrochim Acta Part A Mol Biomol Spectrosc 214:302–308
Zhang X, Wu D, Zhou X, Yu Y, Liu J, Hu N, Wang H, Li G, Wu Y (2019b) Recent progress in the construction of nanozyme-based biosensors and their applications to food safety assay. TrAC Trends Analyt Chem 121:115668
Zhang SS, Bilal M, Zdarta J, Cui JD, Kumar A, Franco M, Ferreira LFR, Iqbal HMN (2021) Biopolymers and nanostructured materials to develop pectinases-based immobilized nano-biocatalytic systems for biotechnological applications. Food Res Int 140:17
Zhao R, Heng M, Chen C, Li T, Shi Y, Wang J (2021) Catalytic effects of Al2O3 nano-particles on thermal cracking of heavy oil during in-situ combustion process. J Pet Sci Eng. https://doi.org/10.1016/j.petrol.2021.108978
Zhironkin S, Demchenko S, Kayachev G, Taran E, Zhironkina O (2019) Convergent and nature-like technologies as the basis for sustainable development in the 21st Century. In: E3S Web of Conferences. EDP Sciences, p 03008
Zhou M, Hou T, Li J, Yu S, Xu Z, Yin M, Wang J, Wang X (2019) Self-propelled and targeted drug delivery of poly(aspartic acid)/Iron–zinc microrocket in the stomach. ACS Nano 13(2):1324–1332
Zhou Y, Wu X, Zhong X, Reagen S, Zhang S, Sun W, Pu H, Zhao JX (2020) Polymer nanoparticles based nano-fluid for enhanced oil recovery at harsh formation conditions. Fuel 267:117251
Zhu L, Tong L, Zhao N, Li J, Lv Y (2019) Coupling interaction between porous biochar and nano zero valent iron/nano α-hydroxyl iron oxide improves the remediation efficiency of cadmium in aqueous solution. Chemosphere 219:493–503
Download references
Author information
Authors and affiliations.
Department of Engineering, University of Science and Culture, Tehran, Iran
Nikta Shahcheraghi
Faculty of Biological Sciences, Kharazmi University, No.43.South Moffateh Ave., 15719-14911, Tehran, Iran
Hasti Golchin, Zahra Sadri, Forough Borhanifar & Shadi Makani
Faculty of Sciences and Advanced Technologies, Science and Culture University, 1461968151, Tehran, Iran
Yasaman Tabari
You can also search for this author in PubMed Google Scholar
Contributions
All authors have participated in (a) conception and design, or analysis and interpretation of the data; (b) drafting the article or revising it critically for important intellectual content; and (c) approval of the final version. This manuscript has not been submitted to, nor is under review at, another journal or other publishing venue. The authors have no affiliation with any organization with a direct or indirect financial interest in the subject matter discussed in the manuscript.
Corresponding author
Correspondence to Nikta Shahcheraghi .
Ethics declarations
Conflict of interest.
On behalf of all authors, the corresponding author states that there is no conflict of interest.
Rights and permissions
Reprints and permissions
About this article
Shahcheraghi, N., Golchin, H., Sadri, Z. et al. Nano-biotechnology, an applicable approach for sustainable future. 3 Biotech 12 , 65 (2022). https://doi.org/10.1007/s13205-021-03108-9
Download citation
Received : 28 July 2021
Accepted : 30 December 2021
Published : 09 February 2022
DOI : https://doi.org/10.1007/s13205-021-03108-9
Share this article
Anyone you share the following link with will be able to read this content:
Sorry, a shareable link is not currently available for this article.
Provided by the Springer Nature SharedIt content-sharing initiative
- Nanotechnology
- Nano-biotechnology
- Nanomaterial
- Nano-therapies
- Find a journal
- Publish with us
- Track your research
- Bibliography
- More Referencing guides Blog Automated transliteration Relevant bibliographies by topics
- Automated transliteration
- Relevant bibliographies by topics
- Referencing guides
Technology articles within Nature Nanotechnology
Comment | 02 October 2023
Navigating advanced technology transitions: using lessons from nanotechnology
As researchers, developers, policymakers and others grapple with navigating socially beneficial advanced technology transitions — especially those associated with artificial intelligence, DNA-based technologies, and quantum technologies — there are valuable lessons to be drawn from nanotechnology. These lessons underscore an urgent need to foster collaboration, engagement and partnerships across disciplines and sectors, together with bringing together people, communities, and organizations with diverse expertise, as they work together to realize the long-term benefits of transformative technologies.
- Andrew D. Maynard
- & Sean M. Dudley
Comment | 06 June 2023
Developing trends in nanomaterials and their environmental implications
Nanotechnology is advancing at an accelerated pace in applications and novel nanomaterials. To become an enabling technology for a more sustainable society, we identify and assess nanomaterials and applications trends with potentially significant environmental implications.
- Arturo A. Keller
- , Alex Ehrens
- & Bernd Nowack
Comment | 17 March 2023
mRNA therapy at the convergence of genetics and nanomedicine
Since the early 1990s, the intersection of genetics and nanomedicine has found a home in the clinic as one of the game changers of the past decade, holding great promise in fighting diseases by rapidly developing much-needed therapeutic platforms, from cancer to infectious or genetic diseases. And this revolution was just triggered by the amazing evolving world of messenger RNA and its ‘cues’.
- , Robert Langer
- & José Rueff
Comment | 21 April 2022
Rapid response through the entrepreneurial capabilities of academic scientists
Academic scientists who develop entrepreneurial capabilities can make strategic, path dependent decisions that enable university spin-offs to rapidly respond to global crises.
- Andrew Park
- , Azadeh Goudarzi
- & Elicia Maine
Correspondence | 25 November 2020
Advances and challenges towards consumerization of nanomaterials
- Steffen Foss Hansen
- , Oliver Foss Hessner Hansen
- & Maria Bille Nielsen
Editorial | 06 July 2018
Factors that make an impact
Number of citations in academic papers is not always a good measure for the influence of applied research papers.
Q&A | 06 July 2018
From an idea to a technology
Nature Nanotechnology has asked Jong-Hyun Ahn, Yi Cui and Hagan Bayley, corresponding authors of the three papers published in the journal that have received the highest number of citations in the patent literature, to share their insights about doing applied research in academia and whatit takes to transform an idea into a viable technology.
- Alberto Moscatelli
Analysis | 07 May 2018
A critical evaluation of nanopesticides and nanofertilizers against their conventional analogues
Analysis of existing data on nanofertilizers and nanopesticides show that they have a gain in efficacy with respect to conventional products, but more data are necessary to evaluate all risks and benefits of using these materials in agriculture.
- Melanie Kah
- , Rai Singh Kookana
- & Thomas Daniel Bucheli
Commentary | 06 September 2017
A cautionary note on graphene anti-corrosion coatings
Despite graphene's apparent potential for anti-corrosion coatings, it is cathodic to most metals and can promote corrosion at exposed graphene–metal interfaces. This may accelerate dangerous localized corrosion that can seriously weaken the coated metals.
- Chenlong Cui
- , Alane Tarianna O. Lim
- & Jiaxing Huang
Editorial | 06 December 2016
Nanotech in motion
Feature | 06 December 2016
An electrifying journey
Formula E — an all-electric car race series — provides a platform for displaying new technologies, and potentially accelerating their development.
- Andreas Trabesinger
Article | 01 August 2016
Improving the efficacy and safety of biologic drugs with tolerogenic nanoparticles
Synthetic nanoparticles containing rapamycin — a common immunosuppressant drug — when co-administered with any free antigen can induce immune tolerance, offering a way to rescue novel drugs that have failed in the clinic due to antidrug antibodies.
- Takashi K. Kishimoto
- , Joseph D. Ferrari
- & Roberto A. Maldonado
Thesis | 03 March 2016
Navigating the risk landscape
The potential risks surrounding nanotechnology can often appear complex and confusing. But with some basic guideposts, argues Andrew D. Maynard , navigating them can become a little easier.
Commentary | 03 March 2016
Long-term monitoring for nanomedicine implants and drugs
Increasing globalization means that traditional occupational epidemiological approaches may no longer apply, suggesting a need for an alternative model to assess the long-term impact of nanomaterial exposure on health.
- Michaela Kendall
- & Iseult Lynch
Thesis | 03 February 2016
Towards a new scale
Work on a new technology roadmap and an exceptional wave of consolidation hint at fundamental changes in the micro- and nanoelectronics industry, as Christian Martin explains.
- Christian Martin
Letter | 11 January 2016
Tailoring high-temperature radiation and the resurrection of the incandescent source
A nanophotonic radiation interference system transforms a tungsten filament into a highly efficient thermal emitter for lighting applications.
- Ognjen Ilic
- , Peter Bermel
- & Marin Soljačić
Thesis | 07 January 2016
Less is Moore
Predictions for the development of microelectronics provide a valuable example about the virtues of measured promises in nanotechnology, as Chris Toumey explains.
- Chris Toumey
Letter | 14 December 2015
Plasmonic colour laser printing
Pulsed laser light locally melts nanostructured elements of a plasmonic metasurface to create coloured pixels with a resolution up to 127,000 dots per inch (DPI).
- Xiaolong Zhu
- , Christoph Vannahme
- & Anders Kristensen
Thesis | 03 December 2015
Navigating the fourth industrial revolution
Andrew D. Maynard considers the challenges of ensuring the responsible development and use of converging technologies.
Research Highlights | 06 October 2015
Start-ups: How to be an entrepreneur scientist
- Ai Lin Chun
Our choice from the recent literature
Thesis | 03 September 2015
Why we need risk innovation
If emerging technologies such as nanotechnology are to reach their full potential we need to radically change our approach to risk, argues Andrew D. Maynard .
Thesis | 05 February 2015
The (nano) entrepreneur's dilemma
Emerging technologies need to be developed responsibly if their benefits are to outweigh any potential risks. Yet do entrepreneurs really have the luxury of grappling with future consequences from the get-go, asks Andrew D. Maynard .
In the Classroom | 05 February 2015
Remodelling technology transfer
Should inventors control the fate of their own inventions? In the US, most universities think not. But, as Emmanuel Dumont explains, the Jacobs Technion-Cornell Institute at Cornell Tech in New York City bets otherwise.
- Emmanuel L. P. Dumont
Thesis | 07 May 2014
Driving change in the battery industry
High-capacity silicon anodes could improve the performance of lithium-ion batteries for electric vehicles, but their cyclability has been limited. Christian Martin analyses recent progress in nanoscale engineering that addresses this shortcoming.
Thesis | 05 February 2014
Binary challenge
Since the 1960s, improvements in integrated circuit design and processing have generated exceptional growth in the semiconductor industry. With feature sizes approaching a few nanometres and 450-mm-wide wafers looming, nanoelectronics is now facing its defining years, says Christian Martin .
Browse broader subjects
- Business and industry
Quick links
- Explore articles by subject
- Guide to authors
- Editorial policies

The Ohio State University
- BuckeyeLink
- Search Ohio State

Professor Ahmad Umar ranks among most cited scientists in the world
He believes in shaping the future of nanomaterials by nurturing the next generation of scientists and engineers through lecturing, grant writing and collaboration on campus. They confirm his mission to exceed scientific boundaries.
My time at Ohio State University has been incredibly rewarding. Surrounded by supportive colleagues and state-of-the-art facilities, has allowed me to thrive in the collaborative atmosphere fostered by the university. I am grateful for the opportunity to work alongside talented individuals who share his passion for research and education. Professor Ahmad Umar
He is one of the founders of Advanced Materials and Nano-Research Centre at Najran University and is the Director for STEM pioneers training lab. He has received many international awards and is an editor and editor-in-chief for several scientific journals.
- Share via Facebook (link opens in new window)
- Share via Twitter (link opens in new window)
- Share via LinkedIn (link opens in new window)
.cls-1{fill:#a91e22;}.cls-2{fill:#c2c2c2;} double-arrow Ahmad Umar
Related news.

An official website of the United States government
The .gov means it’s official. Federal government websites often end in .gov or .mil. Before sharing sensitive information, make sure you’re on a federal government site.
The site is secure. The https:// ensures that you are connecting to the official website and that any information you provide is encrypted and transmitted securely.
- Publications
- Account settings
Preview improvements coming to the PMC website in October 2024. Learn More or Try it out now .
- Advanced Search
- Journal List
- v.12(3); 2022 Mar

Nano-biotechnology, an applicable approach for sustainable future
Nikta shahcheraghi.
1 Department of Engineering, University of Science and Culture, Tehran, Iran
Hasti Golchin
2 Faculty of Biological Sciences, Kharazmi University, No.43.South Moffateh Ave., 15719-14911 Tehran, Iran
Zahra Sadri
Yasaman tabari.
3 Faculty of Sciences and Advanced Technologies, Science and Culture University, 1461968151 Tehran, Iran
Forough Borhanifar
Shadi makani.
Nanotechnology is one of the most emerging fields of research within recent decades and is based upon the exploitation of nano-sized materials (e.g., nanoparticles, nanotubes, nanomembranes, nanowires, nanofibers and so on) in various operational fields. Nanomaterials have multiple advantages, including high stability, target selectivity, and plasticity. Diverse biotic (e.g., Capsid of viruses and algae) and abiotic (e.g., Carbon, silver, gold and etc.) materials can be utilized in the synthesis process of nanomaterials. “Nanobiotechnology” is the combination of nanotechnology and biotechnology disciplines. Nano-based approaches are developed to improve the traditional biotechnological methods and overcome their limitations, such as the side effects caused by conventional therapies. Several studies have reported that nanobiotechnology has remarkably enhanced the efficiency of various techniques, including drug delivery, water and soil remediation, and enzymatic processes. In this review, techniques that benefit the most from nano-biotechnological approaches, are categorized into four major fields: medical, industrial, agricultural, and environmental.
Introduction
The development process of a sustainable future generally consists of methods that ensure the satisfaction of future needs, while fulfills the current generation’s requirements (Raghav et al. 2020 ). To obtain a proper overview of upcoming demands in the future, it is important to anticipate future stressors (e.g., climate change) (Iwaniec et al. 2020 ). Since nanotechnology is applicable in various majors, it is expected that nano-based techniques will take a key role in a sustainable future (Raghav et al. 2020 ), along with making substantial impacts on the universal economic situation due to their wide range of applications in variant industries (Adam and Youssef 2019 ). The unification of diverse fields in science, Inspired by the oneness of nature, is one of the most noticeable subject matters now in the early twenty-first century. Merging four massively operational fields of science has received great attention in recent decades: nanotechnology, biotechnology, information technology, and cognitive sciences (NBIC), which are known as “convergent technologies” (Roco and Bainbridge 2013 ). Non-renewable sources don’t seem efficient for providing large amounts of energy required in various industrial technologies. Convergent technologies are considered as a remedy for this issue. For instance, several nano-based technologies, which consume biological-renewable energy sources, have been introduced (Zhironkin et al. 2019 ). The unification of material on nanoscale makes the mentioned combination of multiple technologies possible. Hence, nanotechnology plays a critical role in NBIC advancement (Roco and Bainbridge 2013 ). According to the definition set by National Nanotechnology Initiatives in 1999, Nanotechnology is an advanced area of research that allows for the production of a wide class of materials in the nanoscale range (less than 100 nm) to make use of size-and structure-dependent properties and phenomena (Luo et al. 2020 ). Although “nano” is defined as that which is less than 100 nm in size, the use of this definition in the biomedical field is less strict and instead may encompass particles up to 1000 nm in size (Landowski et al. 2020 ). Nanotechnology has a wide range of applications, including Agricultural usages (Ndlovu et al. 2020 ), biofuel production (Zahed et al. 2021a ), cancer Immunotherapy (Goracci et al. 2020 ), carbon capture (Zahed et al. 2021b ) and biomarker detection like nanobiochips, nanoelectrodes, or nanobiosensors (Bayda et al. 2020 ). Nanomaterials (NMs) are chemical substances or materials that are manufactured and used at a very small scale, i.e., 1–100 nm in at least one dimension. NMs are categorized according to their dimensionality, morphology, state, and chemical composition (Saleh 2020 ). NMs can be used for rapid extraction of RNA of the novel coronavirus (Kailasa et al. 2021 ). Expanding nanoscience through various branches can eventually enhance the intelligence and capability of individuals, solve various social issues, cure numerous diseases, and generally improve the quality of mankind's life in the long term (Roco and Bainbridge 2013 ). Deploying nanotechnology into biotechnology will help the commercialization process of nano-based techniques and make them more practical in the industry (Maine et al. 2014 ). The idea of developing interdisciplinary research (IDR) (Jang et al. 2018 ) in science presents a promising landscape of the future, in which human intelligence has reached such high levels that the term “superhuman” would be more proper for humankind. According to the Israeli philosopher Harari, with the appearance of a highly technologically advanced society, only individuals with great intelligence and technological advancements can survive through natural selection in society. He states that superhumans will be produced by society eventually, considering the logic of social Darwinism, and this will be a remarkable phenomenon of the twenty-first century (Mantatov et al. 2019 ). One massive application of nanobiotechnology is enhancing the efficiency of various therapies (Table (Table1). 1 ). The application of nanobiotechnology in delivering chemical drugs or gene modifying agents to their target cells will increase the efficiency of the treatments and reduce the side effects remarkably. Within the previous two decades, RNA-based therapeutic methods, including messenger RNA (mRNA), microRNA, and small interfering RNA (siRNA), have been supremely developed. These therapeutic approaches are expected to be operative in the treatment and prevention of various diseases, such as cancers, genetic disorders, diabetes, inflammatory diseases, and neurodegenerative diseases (Lin et al. 2020 ). In the case of cancers, conventional therapies (surgery, chemotherapy, and irradiation) may cause severe side effects to patients, plus they are often inefficient for disease treatment (Hager et al. 2020 ). Loading anti-cancer drugs into nanomaterials provides a nano-based drug delivery system that detracts the side effects. Platinum (Pt) compounds are one of the most common anti-cancer drugs since 1978. Pt drugs directly aim at the DNA of the targeted cells, thus covering up the defects of the malformed DNA repair mechanisms in cancerous cells. Encapsulating Pt drugs into liposomes constructs a nano-based drug delivery system for treating cancers (Rottenberg et al. 2021 ). Gold nanoparticles (AuNPs) are advantageous options for cancer treatment and diagnosis. AuNPs are created in the size range between 1 and 150 nm and in various shapes, including nanorods (AuNRs), nanocages, nanostars, and nanoshells (AuNSs). AuNPs consist of high rates of biocapability and exhibit controlled patterns of medicine release in the drug delivery process. AuNPs consist of conduction electrons on their surfaces which get excited by certain wavelengths of light. This feature enables AuNPs to adsorb light and produce heat that is fatal to cells. Destroying the cancerous cells with the heat released under irradiation is called photothermal therapy (PTT) or photodynamic therapy (PDT) (D’Acunto et al. 2021 ).
Medical applications of nanobiotechnology
On the other hand, RNA-based therapies can regulate the expression of immune-relevant genes, therefore increasing anti-tumor immune responses directly. Several nanomaterials have been introduced that can deliver nucleic acid therapeutics to tumors and immune cells (Lin et al. 2020 ). There are biomimetic strategies for providing a co-delivery system that is capable of supporting both chemical and RNA-based therapies (Liu et al. 2019 ). Considering RNAs as therapeutic agents or drug targets requires precise knowledge about the 3D structure of specific RNAs. There are reliable algorithms for pronging the second structure of RNAs, but the tertiary architecture which determines the RNA’s functions is quite challenging to anticipate. Bioinformatics provides several methods for predicting the tertiary structures of RNAs such as Vfold, iFoldRNA, 3DRNA, and RNAComposer. They all face particular hurdles, but it should be noted that the field of computational RNA structure anticipation, has a bright future (Biesiada et al. 2016 ). RNA-based vaccines are quite impressive immunotherapeutic tools in cancer therapies. However, the in vivo delivery of synthesized mRNAs could face some obstacles. Encrusting mRNAs with a lipid-polyethylene glycol (lipid-PEG) shell increases the mRNA delivery rate up to 95% more than the conventional nanoparticle-free mRNA vaccines (Islam et al. 2021 ).
In RNA-based nano-techniques, utilizing large-sized RNAs faces several difficulties. Wang et al. have reported an interesting method of using gold nanoparticles (enriched by expanded genetic alphabet transcriptions) to increase the effectiveness of detecting the large natural or artificially synthesized RNAs through an RNA nano-based labeling technique. These techniques are highly dependent on the conjugation between nanoparticles and RNAs (Wang et al. 2020 ). Since gene sequencing is of great importance, multiple biotechnology-based diagnostic tools, including quantitative PCR, DNA barcoding, next-generation sequencing, and imaging techniques are commonly currently used. These methods are considered economically advantageous, along with providing a reliable diagnosis. Incorporating nano-based sensors with mentioned tools increases the sensitivity and spatiotemporal resolution, which are two fundamental features of the gene sequencing process (Kumar et al. 2020 ). Designing nano-based devices for diagnosis of severe acute respiratory syndrome coronavirus 2 (SARS-CoV 2) has been promoted recently. Nanomaterials such as gold nanoparticles, magnetic nanoparticles, and graphene (G) significantly increase the accuracy and decrease the required time and costs. Hence, render beneficial tools for viral detection more effective compared to the traditional techniques. Nanoparticles are specified via anti-bodies to identify particular antigens on the surface of the virus. Suspected samples from the patient, air, and surface can get examined by nano-based serological or molecular diagnosis methods (Abdelhamid and Badr 2021 ).
Nanomaterials can be utilized in the form of membranes. Chemically or physically synthesized nanomembranes remarkably advance the conventional water purification techniques (Lohrasebi and Koslowski 2019 ; Kim et al. 2020 ). Incorporating nanomembranes with bioreactors is the basis of the membrane bioreactor (MBR) technique, which is exploited in wastewater reclamation (Ma et al. 2018 ). Eliminating pollutant components from the environment is one of the main purposes of nanobiotechnology (Table (Table2). 2 ). In the agricultural fields, nano-bio technologically modified pesticides and fertilizers notably prevent crop loss. Nano-based bioremediation processes have been developed to reduce soil pollutions and are expected to improve both environmental and agricultural approaches (Usman et al. 2020 ). Several studies are expanding the idea of producing nano plants that show better biological performances (e.g., photosynthesis) compared to natural plants (Marchiol 2018 ) (Table (Table3). 3 ). Enzymes empowered by nanomaterials have rendered higher recovery and productivity rates and thus are potentially able to act spotless in different industrial techniques (Adeel et al. 2018 ; Zhang et al. 2021 ) (Table (Table4 4 ).
Environmental applications of nanobiotechnology
Agricultural applications of nanobiotechnology
Industrial applications of nanobiotechnology
The objective of this study is to review the applications of nanoscience in enhancing the efficiency of biotechnological methods (Fig. 1 ).

Diverse Applications of Nanobiotechnology: multiple techniques, including Drug delivery-based therapies, remediating processes, and industrial nano-bio catalysts benefit from nano-scaled particles
Application of nano-based materials for drug delivery, therapeutic and diagnostic processes
One recently promoted technique in the gene therapy field is the application of the CRISPR/Cas9 systems, which has been indicated to be highly effective in the treatment of monogenic disorders, non-monogenic disorders, and infectious diseases. Emerging studies have suggested that nanocarriers, which are created from Polymer polyethyleneimine (PEI), are more efficient in delivering CRISPR/Cas9 systems to targeted cells compared to the viral carriers (Deng et al. 2019 ). Gene mutation-related diseases such as cancers and human immunodeficiency viruses are potentially treated by DNA-based vaccines. This type of vaccine enhances disease symptoms by delivering specific gene sequences-which are embedded in plasmids- to targeted cells. Despite having clinical utilization, DNA vaccines face limitations in delivering their genetic cargos to the target cells. Designing efficient nano-delivery systems will eliminate such deficiencies PEI (Lim et al. 2020 ). Virus-like nanoparticles (Jeevanandam et al. 2019 ) seem to form applicable nanocarriers for this purpose (Fig. 2 ).

Encapsulating therapeutic agents within nanoparticles: embedding medicine or gene-modifying agents into the nanoparticles remarkably enhances the therapeutic efficiency along with diminishing potential side effects
Nanomaterials used in cancer diagnosis can be mainly divided into contrasting agents (magnetic, iron oxide and gold nanoparticles) and fluorescent agents (quantum dots). Some nanocarriers have inherent optical properties (such as carbon nanotubes, gold and magnetic nanoparticles) that can be converted into high energy to cells for destruction and can serve as nanotheranostics (Barani et al. 2021 ).
Nanomaterials used in smart drug delivery-based cancer therapies are categorized as organic and inorganic materials. Micelles, vesicles, multilamellar liposomes, and solid lipid nanoparticles are some examples of self-assembled organic nanomaterials. Other organic materials are not capable of self-assembling and need to be synthesized, such as nanotubes and dendrimers. Gold nanoparticles, quantum dots, mesoporous silica nanoparticles, and superparamagnetic iron oxide nanoparticles (SPIONs) are classified as inorganic nanomaterials (Lombardo et al. 2019 ). SPIONs are vastly utilized in therapeutic approaches, including cancer therapy, radiation therapy, and tissue engineering. SPIONs are synthesized through different physical, chemical, and biological methods. Bacteria and plants are the biomaterials upon which the biological method is based (Samrot et al. 2020 ). Nanoparticles containing both organic and inorganic materials (hybrid nanoparticles) have been indicated to be highly efficient, as well (Lombardo et al. 2019 ). Embedding targeting ligands (e.g., antibodies, peptides, aptamers, and small molecules) on the surface of nanoparticles assures the delivery of medicines to specific sites in the body, such as tumor tissues. The mentioned process is called: “targeted drug delivery system” (Doroudian et al. 2021 ). There are two types of targeting delivery: passive targeting and active targeting. In the passive form, the high aggregations of medicines at the tumor sites are related to the nano-scaled size of the nanocarriers. The tight junctions between epithelial cells of the vessel tissues prevent the nanoparticles from exiting the vessel. The cancerous cells loosen the tight junctions of the adjacent vessels. Therefore, nanocarriers can pass through the vessel and get into the tumor site. The targeting ligands incorporated with nanoparticles are not responsible for the passive targeting action. The binding between the targeting ligands and the particular receptors on the cancerous cells-which are exclusively found on the surface of the tumor cells- causes a more precise drug delivery, which is known as active targeting (Doroudian et al. 2019 ). Although drug-loaded nanoparticles efficiently carry the medicines to target cites, according to the in-vivo studies, these nanoparticles might not be quite biodegradable. Hence using such nanoparticles could lead to toxicities and side effects. It is worth mentioning that Zhou et al. have developed biodegradable nanoparticles using poly (aspartic acid) (PASP) microtube, a thin Fe intermediate layer, and a core of Zn (Zhou et al. 2019 ).
Nano-based drug delivery systems provide highly promising prospects for treating neurodegenerative disorders. It is reasonable to assume that treating neurological diseases by conventional drug delivery systems is extremely challenging due to the presence of the blood–brain barrier (BBB). The blood–brain barrier prevents the entrance of therapeutical agents to the central nervous system (CNS), therefore, making the conventional therapies inadequate. The blood–brain barrier provides a stable environment for the CNS and regulates the cell-to-cell interactions, which take place in the CNS. The dysfunction of the blood–brain barrier leads to severe neurodegenerative disorders (e.g., Parkinson’s disease (PD), Alzheimer’s disease (AD), amyotrophic lateral sclerosis (ALS), and multiple sclerosis (MS)). The blood–brain barrier is responsible for the proper functioning of the CNS, so naturally, it has a super-sensitive permeability. This feature of the blood–brain barrier is highly related to the tight junctions between the barrier’s cells. Only 1–4 percent of most CNS medicines succeed in passing the blood–brain barrier. Nanoparticles are more likely to pass the barrier because of their nano-scaled size. Encapsulating drugs in nanoparticles can significantly increase the drug transmission rate through the blood–brain barrier (Furtado et al. 2018 ). For instance, graphene, metals, carbon-nanotubes, and metal-oxides are the nanomaterials that can get exploited in the treatment procedure of patients with Alzheimer’s disease (AD). AD is caused by different genetic and environmental cues. Chemical and electrical malformations are observed in the brain of an AD patient. Acrine and physostigmine, which are conventional medicines for AD, have been proved to stimuli severe effects on the gastrointestinal tract and nervous system. Therefore, attention is drawn to nano-based therapies (Nawaz et al. 2021 ). Marcos-Contreras et al. have proposed that the augmentation of VCAM-1 ligands to the drug-loaded nanocarriers can significantly improve the cerebral accumulation rate of nanoparticles in inflamed brains (Marcos-Contreras et al. 2020 ) (Fig. 3 ).

Nano-based drug delivery in the therapies of neurodegenerative disorders: blood–brain barrier (BBB) is a noticeable obstacle for conventional medicines; however, drugs encapsulated within nanoparticles efficiently penetrate through the BBB and reach the central nervous system (CNS)
Although nano-based medications of neurodegenerative disorders seem spotless theoretically, the internal environment of the body puts out several obstacles on the path of the medicine nano-delivering. For instance, lipid nanoparticles (LNPs) may safely carry their therapeutic cargos to the targeted cells, but if the drug needs to reach the cytoplasm, lipid nanoparticles are not capable of efficiently crossing the cell membrane. Small interfering RNAs (siRNAs) are delivered to hepatocytes via lipid nanoparticles, but only 2% of them accomplish reaching to the cytoplasm. It should be mentioned that big data and computational methods can help scientists to predict the in-vivo challenges of nano-drug delivery to design proper techniques to overcome them (Paunovska et al. 2019 ). Besides, bioinformatics provides tools for measuring the interaction rate between exploited nanomaterials and drug targets (Nawaz et al. 2021 ). Designing efficient nanomaterials is fundamental for nanotechnological approaches. Carbon nanotubes (CNTs) and graphene-based nanomaterials have been vastly utilized in nanotechnology during the last two decades (Kinloch et al. 2018 ). As a case in point, Single-walled carbon nanotubes (SWCNTs) are considered as excellent options for designing nano-based biomedical approaches, including but not limited to drug delivery systems. The most noticeable features of SWCNTs are their great photophysical properties (Farrera et al. 2017 ). Even though Carbon nanotubes (CNTs) and graphene-based nanomaterials have unique qualities such as high flexibility, they face some challenges in their load transfer capability, dispersion, and viscosity. Hence, creating more applicable and eco-friendly nanomaterials has drawn intense attention (Kinloch et al. 2018 ). AlNadhari et al. have introduced algae as a green and eco-friendly source of materials that can be used in nanoparticles. Algae-based nanoparticles in the biomedical field consist of therapeutical characteristics, such as antibacterial, anti-fungal, and anti-cancer features (AlNadhari et al. 2021 ). Milk-derived proteins such as β-lactoglobulin (β-LG), lactoferrin (LF), and the caseins (CN) are other biological alternatives for synthesizing nanocarriers. Anti-cancer medicines have been embedded into protein-based nanocarriers and successfully deteriorated cancerous tumors (Tavakoli et al. 2021 ). Azarakhsh et al. have demonstrated specific binding sites for the anti-cancer drug, Oxali-palladium (OX) and iron nanoparticles (NP) on the Beta-Casein (β-CN). Hence, the Beta-Casein can perform as an efficient carrier for both agents (Azarakhsh et al. 2021 ). One common strategy in designing nanocarriers for cancer therapies is to create nanoparticles that can detect the vitamin or growth factor receptors on target cells. Cancerous cells usually over-express the receptors for such nutrients so that they can keep their high proliferation rate (Peer et al. 2020 ). Reprogramming the nutrient signaling and micropinocytosis of the cancer cells seriously affects the efficacy of Nano-particulate albumin-bound paclitaxel (nab-paclitaxel, nab-PTX); which is one of the most commonly prescribed nanomedicines (Li et al. 2021 ).
Antimicrobial peptides (AMPs) are short-chain, often cationic, peptides possessing several attributes which make them attractive alternatives to conventional antibiotics with s a low likelihood of resistance developing in target organisms (Meikle et al. 2021 ). Conjugation and functionalization of nanoparticles with potentially active antimicrobial peptides has added advantages that widen their applications in the field of drug discovery as well as a delivery system, including imaging and diagnostics (Mohid and Bhunia 2021 ).
Silver nanoparticles coated with zinc oxide (Ag@ZnO), can stimulate proliferation and migration of human keratinocytes, HaCaT, with increased expression of Ki67 and vinculin at the leading edge of wounds. Interestingly, Ag@ZnO stimulates keratinocytes to produce the antimicrobial peptides hBD2 and RNase7, promoting antibacterial activity against both extracellular and intracellular Staphylococcus aureus isolated from wounds (Majhi et al. 2021 ).
Wound dressing is an important action against an injury. In recent years, nanotechnology has been combined with wound dressing techniques, and there are several new materials and techniques available for this action. The nanoparticles’ dimensions make them suitable for penetrating into the wound. Thus, bioactive agents and drugs can be released locally (De Luca et al. 2021 ). Numerous synthetic and natural materials have been applied for wound healing; Hyaluronic Acid, as an illustration, is one of the most-used materials (Ahire and Dicks 2016 ).
In 2017 Polyethylene Oxide (PEO)-hyaluronic acid (HA) nanofibers as an inhibitor of Listeria monocytogenes infection (Ahire et al. 2017a ). Gauze is a traditional wound dressing used to protect dermal wounds from bacterial infection. In a study in 2021, an antibacterial gauze was prepared by the combined use of antimicrobial peptides and AgNPs. The prepared antibacterial gauze showed excellent antibacterial activity against E.coli, S. enteritidis, S. aureus , and B. cereus and also exhibited good biocompatibility (Chen et al. 2021a , b ). In 2014, Ahire and Dicks introduced 2,3-Dihydroxybenzoic Acid-Containing Nanofiber as a suitable nanomaterial for wound dressing as it prevents Pseudomonas aeruginosa infection (Ahire and Dicks 2014 ). To inhibit the growth of this microorganism, Copper-Containing Anti-Biofilm Nanofiber Scaffolds can be used too. Copper-containing nanoparticles have the potential of inhibiting Escherichia coli growth either (Ahire et al. 2016 ). Surfactin-loaded nanofibers are also a great candidate to be used in wound dressings or in the coating of prosthetic devices to prevent biofilm formation and secondary infections (Ahire et al. 2017b ). In addition to nano-therapies, nano-diagnostic agents- metal nanoparticles- have been indicated to be highly applicable in the detection of viruses, including covid-19 (Fouad 2021 ). Several biotic [e.g., algae (AlNadhari et al. 2021 ) and viral capsid (Jeevanandam et al. 2019 )] and abiotic [e.g., gold, silver, graphene oxide, and zin oxide (Fouad 2021 )] nanomaterials have been reported to be applicable in biomedical processes. The combination of biotic and abiotic sources provides efficient nanomaterials as well. For example, the highly effective graphene-starch nanocomposites, are resulted from embedding graphene-based nanomaterials into the starch biopolymers (Mishra and Manral 2021 ). The delivery of therapeutics via nanoemulsions (NE) has shown striking results. Sánchez-Rubio et al. have successfully defeated deficiencies of vitamin E (e.g., hydrophobicity and low stability) by creating nanoemulsions comprising vitamin E. the sperm samples derived from the red deer’s epididymal tissue was treated with the mentioned nanoemulsions and the sperms’ viability and resistance against oxidative stress, was increased (Sánchez-Rubio et al., 2020 ). Jeong et al. have reported another growth-promoting method that elevates the maturation process of cultured cells. The mentioned technique aims to develop an extremely operational and cost-effective bioreactor that enables in-vitro maturation of heart tissue. Next-generation stage-top incubator (STI) containing nano grooves patterned PDMS diaphragm (NGPPD) was designed to boost cell maturation and myogenic differentiation. The surface of NGPPD was covered with a slim layer of gold (Au) (Jeong et al. 2021 ). Microfluidic systems are proven to have applications in biological analysis, tissue engineering, etc. Embedding nanolitre volumes into micro-sized fluidic channels is the basis of the aforementioned technique (Valencia et al. 2020 ).
Application of nanoparticles on bioreactors as contributory agents
Since wastewater reclamation is a universal challenge and plays a major role in providing clean water for many people across the world, various techniques have been developed for this purpose. Among them, the application of membrane bioreactors (MBRs) in water purification has attracted great attention recently. In the MBR technique, the conventional activated sludge (CAS) process is incorporated with a filtration process provided by a physicochemical membrane (Ma et al. 2018 ). It has been shown that treating the mentioned membrane with nanoparticles in different types of MBR techniques can significantly improve the efficiency of the process (Abass and Zhang 2020 ; Jiang et al. 2019 ). The pharmaceutical industry produces one of the most pollutant wastewaters; which contains various amounts of organic compounds, including benzene, polynuclear aromatic hydrocarbons (PAHs), and heterocyclic, etc. these compounds have high Chemical Oxygen Demand (COD) and low degradability; which makes conventional biological treatments inefficient for treating them. However, applying O 3 , O 3 /Fe 2+ , O 3 /nZVI (nano zerovalent iron) processes in wastewater purgation has made noticeable signs of progress. Nano catalytic ozonation process (O 3 /nZVI) in a semi-batch reactor has the highest effect on advancing degradation amongst all (Malik et al. 2019 ). An experiment conducted in southern Tehran succeeded in removing the Methyl Tertio Butyl Ether (MTBE) and benzene from groundwater, using Fenton’s chemical oxidation with stabilized nano zerovalent iron particles (S-NZVI) as a catalyst. The removal efficiency of MTBE and benzene were increased to 90% and 96%, respectively, by reducing the pH of the reaction environment down to 3.2. Acidification of the environment decreased iron consumption as well (Beryani et al. 2017 ).
Nano-bioremediation
One green and cost-effective approach for treating the pollutant soils to reduce their toxicity is applying living organisms (bacteria, fungi, plants, etc.) through a process named: “bioremediation.” Integrating bioremediation with nanoparticles increases the efficiency of the process (Usman et al. 2020 ). The technology of nano-remediation is a sustainable method to reduce the contaminants of the soil by various means (Yue et al. 2021 ; Sajjadi et al. 2021 ; Lian et al. 2021 ). As an example, the reduction of Cr (VI) levels using this technology is known to be worthwhile in many aspects (Azeez et al. 2020 ; He et al. 2020 ). Chemically active nanoparticles can trigger the dechlorination/dehalogenation process in organic pollutants and neutralize them, consequently. Even the toughest pollutants are targeted in this nano-bio-based remediation method. The time needed for the purgation of highly contaminated soils will be minimized by virtue of the mentioned technique (Usman et al. 2020 ). Iron oxide nanoparticles (NP) and Fe 3 O 4 /biochar nanocomposites are vastly exploited in the synthesis of nanoparticles of nano-bioremediation (Patra Shahi et al. 2021 ). It is worth noting that nano zerovalent iron (nZVI) is an effective technology in the case of remediation that has been applied broadly in recent years due to high levels of reactivity for contaminants (Luo et al. 2021 ; Visentin et al. 2020 ; Ken and Sinha 2020 ; Hou et al. 2019 ; Zhu et al. 2019 ).
The bioremediation process can be used in water purification as well. Separating solid components from liquid waste is a necessary stage in the water remediation process. The fresh market waste may contain infectious components, which can seriously harm humans and plants. Hence, it is important to develop methods to collect, separate, and treat these adverse agents. Solid wastes in the wastewater contain high amounts of carbohydrates and proteins, and they provide matrices for the colonization of infectious organisms. Altogether, the presence of solid wastes improves the growth rate of pathogenic organisms. After solid matters got collected, they should be stored and treated immediately. The treatment process must not be delayed because the enriched environment of the solid wastes can easily get corrupted. One way to treat them is through triggering the fermentation and composting processes. Adding effective microorganisms (EM), such as lactic acid/phototropic bacteria and yeast, accelerates the conventional fermentation and composting processes used for the solid waste treatment (Al-Gheethi et al. 2020 ). Costa et al. have sequenced the whole genome of the strain Streptomyces sp. Z38, and detected growth-promoting, heavy metal-eliminating, and anti-microbial features within specific biosynthetic genes. Streptomyces sp. Z38 seems to be a suitable agent for bioremediation due to its ability to decompose heavy metals such as Cr (VI) and Cd (II). Costa et al. have supplemented the bioactive water (BW) extracted from Streptomyces sp. Z38 with AgNO 3 additives and produced silver nanoparticles (AgNPs) that are capable of performing the bioremediation process (Costa et al. 2020 ). There are other effective nanomaterials exploited to reduce many pollutants from soil and wastewater. For instance, utilization of nano-manganese oxide to eliminate ZnII/CoII from water (Mahmoud et al. 2020 ), application of nano-semiconductors on water and their Photocatalytic effectiveness (Oliveira et al. 2021 ), nano-scaled Iron (II) sulfide exploited to reduce hexavalent chromium from soil (Tan et al. 2020 ), production of nanocomposite for eliminating viruses (Al-Attabi et al. 2019 ), and successful application of nano biosurfactants which cause no toxicity for the environment (Debnath et al. 2021 ). Nano-bioremediation as an emergent approach causes some concerns and benefits at the same time. It is possible that nanomaterials exploited in this method would be a threat to the organism populations that exist naturally in water bodies. On the other hand, new living organisms would be introduced through bioremediation. The mentioned two scenarios can potentially put the anthropogenic features of ecosystems in danger (Weijie et al. 2020 ). Concerning this problem, however, scientists are trying to apply new methods to remove nanoparticles from marine ecosystems via other technologies (Ebrahimbabaie et al. 2020 ).
Designing nano-based water purification techniques, to overcome the problem of lack of clean water, across the world
Waterborne diseases that cause almost 10–20 million deaths annually are considered crucial health-related issues. According to the World Health Organization and environmental protection agencies, the pollution level of several water bodies has long crossed the defined limitations. Thus, developing methods for purging water from adverse components is of great concern (Sahu et al. 2021 ). The water purification process profits extremely from nanobiotechnology. Nanoparticles are extremely efficient in eliminating pollutants (e.g., dye components) due to their nano-scaled size and increased surface areas. In the case of dye removal, magnetic nanoparticles have been proved to be proper candidates (Lohrasebi and Koslowski 2019 ). Nanoadsorbents such as silica gel, activated alumina, clays, limestone, chitosan, activated carbon, and zeolite are cost-effective and profitable options for eliminating the contaminating agents during water purification process (Ali et al. 2020 ).
Copper and copper compounds are potent biocides and have been utilized as a disinfectant for centuries due to their anti-microbial properties. It becomes more functional in its nano form and exhibits outstanding synergist, anti-fungal, and anti-bacterial effects (Bashir et al. 2021 ).
Copper nanoparticles have the potential of combination with other materials like Polyacrylonitrile (PAN) nanofibres and Polyethylene Terephthalate Filters to act more beneficial (Ahire and Neveling 2018 ; Nguyen et al. 2021 ).
Metallic nanomaterials, carbon-based nanomaterials, nanocomposites, and dendrimers are four major types of nanomaterials that can be applied in wastewater purgation (Murshid et al. 2021 ). Graphene-based nano-channels, which are inspired by aquaporin channels, have been utilized as water filters and are expected to enhance the water permeability and the salt rejection rate. It is worth noting that the efficiency of these filters can be affected by various factors. For example, it has been indicated that increasing the charges on the channel will decrease the water flow through the channel but, on the other hand, increase the ion rejection rate (Lohrasebi and Koslowski 2019 ). Carbon nanotubes (CNTs) have rendered noticeable results in eliminating the water contaminants, as well (Kutara et al. 2016 ).
The biosafety of water purification via finger-sized unit (FSU) has been certified by cellular and animal tests. In one study, Li et al. loaded 3D printed finger-sized units with prepared wheat straw (WS). To prepare WS for mentioned technique, the carbonized wheat straw (CWS) was adjusted with nano-scaled zinc oxide during an in-situ surface-modification process (CWS/ZnO). The resulted FSU was able to reduce bacteria, organic dyes, and heavy metal ions; therefore, elevating the purification efficiency. Since WS is one of the major agricultural wastes worldwide, applying it in water purification will not only cost very low but will reduce the air pollution which is caused by burning WS in many countries. The WS has a hallow, flexible, and electrical conductor structure. These features make WS a great candidate for enhancing water purification performance (Li et al. 2019 ).
For designing a nano-based filtering membrane, nanoparticles don’t always have to be chemically synthesized or externally applied on the membrane. An emerging study has suggested a top-down approach that uses biomass to provide a functional membrane for the purification of the emulsions. This method can be used massively in cleaning oily waters resulting from industrial or domestic activities. The biomass used in the mentioned technique is wood tissue. The lignin and hemicellulose fractions are removed sectionally, and therefore, a highly porous, flexible, and durable membrane is provided. Since the lignin is removed and there is no hydrophobia left, the resulting wood membrane consists of outstanding water-absorbing and anti-oil properties. The wood-nanotechnology-based membrane shows significant efficiency due to its numerous advantages, including being green, economical, easy to produce, durable, and having selective wettability (Kim et al. 2020 ).
Rezaei et al. have synthesized a flower-shaped ZnO/GO/Fe 3 O 4 ternary nanocomposite through the co-precipitation method, which is considered a rather fast and easy synthesis approach. The mentioned nanocomposite improves the ZnO degradation through a performance with an efficiency that is more than two times greater than the efficiency of the methods using ZnO particles alone. Hence, the ZnO/GO/Fe 3 O 4 ternary nanocomposite seems to be an economical and time-saving approach for wastewater remediation (Rezaei et al. 2021 ).
It is worth noting that the vast uses of nanoparticles in different industrial products increase the risk of the inevitable release of nanoparticles into the environment, and therefore cause some concerns about the potential damages of nanobiotechnology. The urban wastewater seems to be highly exposed to industrial nanoparticles. The high concentrations of nanoparticles in the urban wastewater contaminate the sewage sludge, consequently. Wastewater treatment plants (WWTPs) are currently exploited to remove nanoparticles from wastewater and sewage sludge (Wang and Chen 2016 ). Nanoparticles synthesized and utilized in the industry can end up in marine ecosystems. Nanoparticles are developed from various chemical components such as carbon, silver, gold, and copper, which are potentially hazardous to live organisms. Since nanoparticles are extremely small in size, likely, they will easily enter the bodies of aquatic animals. It has been demonstrated that the accumulation of nanoparticles in the animal’s body can cause severe morphological and behavioral deformities. Genetic materials of cells may undergo various changes as well (Gökçe 2021 ).
FeO ion, which is known as Nanoscale zerovalent iron particles (nZVI), is massively used in the synthesis of nanoparticles applied in wastewater nano-based treatments. Bensaida et al. have shown that combining nZVI with another metal (Cu) enhances the growth of the microbial populations in the wastewater treated with this nZVI\Cu bimetallic nanoparticles (Bensaida et al. 2021 ).
Exploiting nanobiotechnology-based methods in food industry
Nanotechnology-based pharmaceuticals were developed primarily, but wide applications of nanoscience in food and agricultural industries have been introduced as well (Sahani and Sharma 2020 ). Utilizing nanoscience in any stage of the food production process-either cultivation, production, post-harvest processing, or packaging—seems to be lucrative. The application of nano-based methods in the food industry has various advantages, but the most arguable of them would be its impact on shelf life augmentation and spoilage prevention (Bhuyan et al. 2019 ). Since Oxygen is known as an important cause of food spoilage in the food industry, scientists have developed the technology of advanced coatings based on nanotechnology to prevent Oxygen from spoiling the product (Rovera et al. 2020 ). Multiple nanoparticles have the potential to deliver nutritional or antimicrobial components into food materials (Bhuyan et al. 2019 ). It has been reported that nanotechnology is a good option to deliver pesticides and nutrients successfully into the soil and improve the strength and tolerance of products in different stressful situations and reduce the probable contaminations (Ali et al. 2021 ). Among different nanoparticles such as silver, titanium dioxide, and zinc oxide, nanoliposomes are found to be small and have a large surface area which makes them more adhesive to biological tissues- therefore more bioavailable in comparison to others. Nanoliposomes are suitable candidates for creating a delivery system during food preparation. Food provided with the help of nanotechnology is called “Nano food” (Bhuyan et al. 2019 ). Nano foods can perform as therapeutic options. It is interesting to mention a recent study that has proposed exploiting nanoemulsions to convey needed nutrients to gastrectomy patients. These types of patients usually suffer from conditions like anorexia, energy deficit, and malnutrition, which can be treated by efficient nutrition delivery provided by nano food (Razavi et al. 2020 ). As mentioned earlier, in the food preparation process, antimicrobial components can be delivered along with nutritional components via a nano-based delivery system. Polyphenols are great examples of substantial antioxidant and antimicrobial agents in the food industry. Nevertheless, polyphenols have some limitations, including instability, low solubility, inefficient bioavailability, and being drastically susceptible to being degraded. There are several factors that reinforce degradation: Oxygen, light, pH, and interactions between polyphenols and other components in food. Polyphenol-loaded nanoparticles relatively overcome the mentioned obstacles due to their capacity to protect phenolic compounds against degrading processes (Milinčić et al. 2019 ). As a renewable and biodegradable source, starch is a useful polymer that has been applied in different fields such as the pharmaceutical and food industries. Nano-size starch is an advanced material with new abilities in the matter of hydrophobicity and stability (Wang and Zhang 2020 ). In the field of the food industry, there are also many other new methods based on nanotechnology, for instance, designing natural proteins as nano-architectures to deliver nutraceuticals (Tang 2021 ), new strategies for packaging food products by exploitation of the knowledge of nano-biotechnology, and nanomaterials (Reshmy et al. 2021 ; Jogee et al. 2021 ; Tiwari et al. 2021 ), utilization of the nano-delivery techniques to overcome the problems of consuming bioactive ingredients (Hosseini et al. 2021 ; Ozogul et al. 2021 ), producing nanoparticles in the shape of powder using the nanospray driers (Jafari et al. 2021 ), detection of food contaminants by nano-Ag combinations (Yao et al. 2021 ), and even the application of nano-engineering in the field of the beverage industry (Saari and Chua 2020 ).
Nano-bio catalysts; an attempt to remove the barriers of enzymatic bioprocesses in the biotechnology industry
Organic enzymes, which are normally found in nature, have large applications in the biotechnology industry. Since organic enzymes are green and eco-friendly, they are usually preferred to commercially synthesized enzymes. Pectinase is considered to be extremely useful for manufacturing purposes. Pectinase application in industrial bioprocesses covers a large range from clarification of juice/ wine and tea/coffee fermentation to wastewater and industrial waste remediation. All enzymes- regardless of being organic or chemically synthesized- consist of limitations that make their usage challenging. Three major disadvantages of enzymes are inefficient recoverability, operational stability, and recyclability (Zhang et al. 2021 ). Functional nanomaterial-based bio-carriers render a proper environment for the enzymatic immobilization process, therefore facilitating recovery and recycling of enzymes and enhancing the efficiency of bioprocesses in the long run. Accordingly, designing nano-based carriers with these features has been attracted great attention. To achieve this aim, Graphene- immobilized nano-bio-catalysts have been proved to be greatly useful due to the Graphene’s characteristics: electrical, optical, thermal, and mechanical high potency (Adeel et al. 2018 ; Zhang et al. 2021 ).
Nanomaterial-based nanocatalysts are useful in optimizing the biodiesel production process. This ability is related to the features of nano-scaled materials, including crystallisability, high adsorption and storage potential, having catalytic activities, and great stability and durability. Various materials can be used to create nanoparticles for this mean; some examples are metal oxide (calcium, magnesium oxide, and strontium oxide), Magnetic material, and Carbon. Carbon-based nanomaterials consist of multiple types, such as carbon nanotubes, carbon nanofibers, graphene oxide, and biochar.
All examples mentioned above have been proved to be highly effective in increasing the efficiency of the biodiesel synthesizing process and reducing the time and cost required for operating the process without utilizing nanotechnology (Nizami and Rehan 2018 ).
Replacing non-renewable energy sources with renewable ones is a great step in guaranteeing a sustainable future. Various devices, including solar and fuel cells, have been developed for this purpose. Conventional fuel cells are made from metal reactants instead of fossil fuels. They provide an electron circulation, transfer electrons from the substrate to specific electrodes, and eventually produce sustainable energy. The metals used as catalysts in fuel cells (e.g., hydrogen, methane, and methanol) are usually expensive and non-durable. On the other hand, biofuel cells use cost-effective bio-catalysts (e.g., microbes and enzymes) instead of metal catalysts. Despite the mentioned advantages, biofuel cells have one major limitation: the low rate of electron transfer between substrate and electrodes, which is significantly enhanced by supplementing biofuel cells with nanomaterials. Nanomaterials are able to assemble the substrate (e.g., enzymes) with the electrodes. In other words, using them in the structure of electrodes, the electron absorption of electrodes improves- related to the high surface area rate of nanomaterials- therefore, a direct transition of electrons between enzymes and electrodes develops. Silver nanoparticles-Graphene oxide (Ag-GO), Graphite, Carbon-nanotube forest (CNTF), Carbon nanotube (CNT), and Nitrogen-doped hollow nanospheres with large pores (pNHCSs) are the nanomaterials applied in nano- biofuel cells. Respectively, Glucose oxidase (GO x ), Glucose oxidase and Laccase, Fructose dehydrogenase & laccase, Glucose oxidase and laccase, and NADH dehydrogenase form the enzymatic system of each nanomaterial (Sharma et al. 2021 ).
Metal–organic frameworks (MOFs); highly advantageous materials
Porous materials are known to be highly advantageous due to their high absorption and surface areas. Zeolites, activated carbons, and silicas are examples of this family, but the most eminent member among them are Metal–organic frameworks (MOFs). MOFs have features that make them unique for several applications. For example, MOFs show a high absorption rate, which is caused by their high surface areas. Another property of MOFs is their possession of several adjustable microporous channels, which makes it easy to produce different and changeable functional sites through them. The latest feature brings MOFs the shape and size selectivity. By controlling the starting materials and reaction parameters, it is possible to determine the morphology of MOFs (Kinik et al. 2020 ; Jun et al. 2020 ) into various shapes, including granule, pellet, thin-film, gel, foam, paper sheet, monolith, and hollow structures (Kinik et al. 2020 ).
There are two types of MOFs: (1) neutral MOFs and (2) ionic MOFs. Ionic MOFs are able to be used directly in anion purgation processes. For example, one approach for reducing the pollutant anions from the environment is synthesizing a cationic framework along with extra-framework anions. The synthesis of mentioned frameworks occurs by utilizing neutral nitrogen donors. The extra-framework anions will exchange with pollutant anions through an Ion exchange process called: “Anion trapping”.
Anions are extremely abundant in nature. One of the most pollutant and hazardous anions is phosphates. These toxic anions are highly used in pesticides. Other examples of toxic anions, which are considerably frequent in industrial wastes, are the bulky anions. These are the dye molecules exploited in industry. Various diseases like cancers, lung/kidney dysfunction, and brain diseases, including Alzheimer’s, are caused by dangerous anions like those mentioned above. Hence, creating methods that are able to recognize and delete the perilous anions from the environment is one of the most appreciated scientific approaches. MOFs have been proved to be functional for this mean (Desai et al. 2019 ).
Since MOFs have considerable surface areas and modifiable structure—different open metal sites and other functional groups can be introduced into their frameworks—they are suitable options for numerous applications which are generally related to detection and storage. In the case of storage, they exhibit acceptable physical adsorption for CO 2 (one of the major causes of global warming), H 2 (a clean energy source), and Methane (CH 4 ). The ability to adsorb variant components makes MOFs proper for water purification applications. Several toxic and harmful components which are responsible for water contamination, including organic pollutants (like dyes and oils) and heavy metal ions, can be detected, adsorbed, and removed by MOFs. Introducing different chemical groups into MOFs creates different internal interactions, which enable MOFs to detect target molecules functionally. Therefore, they can be used in active centers of catalysts, photocatalysts, and biosensors (Kinik et al. 2020 ).
MOFs-based nanozymes
Nanozymes are classified into two types: (1) natural enzymes that are incorporated with nanomaterials and (2) nanomaterials that exhibit inherent enzymatic features. Exploiting MOFs as nanomaterials in nanozyme structures will produce an emergent form of nanozymes, called: “MOF-based nanozymes”; which have multiple advantages over conventional forms. MOFs provide more catalytic sites, simplify the entrance of small substrate molecules -due to their porous structure-, enhance the substrate exclusivity, and altogether improve the catalytic function of enzymes. MOF-based nanozymes are effective in designing biosensors, biocatalysis, and biomedical imaging techniques. A recent promising application of them is in cancer therapy which reduces side effects significantly (Ding et al. 2020 ).
Agricultural usages of nanobiotechnology
Applying nanobiotechnology in agriculture to improve the agricultural production rate has been of great importance recently. Achieving this purpose will solve several problems related to the universal hunger dilemma. Several nanofertilizers, nano pesticides, and nano-bio sensors have been created, which are able to increase crop value and decrease crop loss caused by agricultural pests (Usman et al. 2020 ). Conventional chemical pesticides and fertilizers can be deteriorative for soil composition and fertility. This happens because chemical residues can target many molecules other than the ones that have been defined as their main targets (Chhipa 2019 ). Besides, pesticides can have ruinous impacts on the microorganisms that naturally exist in the environment and are required for the crop’s growth (Nehra et al. 2021 ). Utilizing nanoparticles can considerably reduce such unwanted events due to the high exclusivity of these particles. Silver, zinc, iron, titanium, phosphorus, molybdenum, and polymer are suitable materials to be used in the structure of agricultural nanoparticles (Chhipa 2019 ). Nanoparticles containing nutrients, fertilizers, and pesticides, can be sprayed externally to the plant. The folium will adsorb the nanoparticles and send them to the soil (Chugh et al. 2021 ).
Another application of nanobiotechnology in diminishing the damages of some traditional pesticides is designing nano-bio sensors that can efficiently detect toxic pesticides. Dichlorvos is one of these toxic pesticides that accumulate in the air, soil, water, and crops; and therefore causes neural, genetical, respirational, and muscular disorders. Dichlorvos-sensitive Nano-biosensors comprise immobilized enzymes embedded in nanomaterials. Acetylcholinesterase (AChE), tyrosinase enzymes, and some others are options for the enzymatic part of the nanodevice. For the nano- matrix section, both organic (carbon, graphene, chitosan, and onion membrane) and inorganic (silver, gold, silica, and Titania) options are available (Mishra et al. 2021 ). Nanomaterials can enhance the remediation process of contaminated soils through distinct abiotic and biotic directions, including the nano-bioremediation process (Usman et al. 2020 ).
Other than improving the functions of existed plants, the possibility of introducing engineered plants with better performances has been discussed recently. The term “plant nano bionics” refers to a pioneering idea of involving nanoparticles in living plants to make their intrinsic functions adjustable. The landscape of this idea is designing engineered artificial photosynthetic systems, enhancing the growth rate of this new type of plant, and many other novel applications which are expected to grow extremely in the years ahead (Marchiol 2018 ).
It is necessary to mention that inorganic nanoparticles that may be found in consumer products, may alter the gut composition and could lead to various gut-related diseases. Thus, there have to be some limitations in nanoparticle agricultural usages (Gangadoo et al. 2021 ; Ghebretatios et al. 2021 ).
Using nanoparticles in cosmetic products
Nowadays, due to special and distinctive physicochemical characteristics, nanomaterials are being vastly used in different industries. Recent studies are focused on applying nano-based technologies to improve the quality of cosmetic products. Nanostructures are about to deliver active ingredients to the skin. For this reason, it is more suitable to use lipid particles that are better adaptable to dermal absorption. The high stability of the combination of nanomaterials and lipid particles with cosmetic components indicates high efficiency. However, the probable risks of this method should not be ignored (Benrabah et al. 2020 ; Khezri et al. 2018 ). Producing nanoparticles using plants (Phyto-metal nano-based particles) is another advantageous method to decrease the toxicity of nanomaterials and their hazardous effects on the body. For this reason, this material is suitable for dermal uses and cosmetic applications (Paiva-Santos et al. 2021 ). Chitosan nanoparticles with better penetrability (Ta et al. 2021 ; Sakulwech et al. 2018 ), Gold and silver nanoparticles with a higher ability to reduce microbial contaminants (Séby 2021 ), Titanium dioxide (TiO 2 ) nanoparticles deposited with yttrium oxide (Y 2 O 3 ) with better attenuation of ultraviolet radiation and less cytotoxicity (Borrás et al. 2020 ), nanoparticles with high uptake of oily components (de Azevedo Stavale et al. 2019 ) are other examples of the efficient application of nanotechnology in the field of cosmetic products.
Since nanoparticles are small in size, they exhibit perfect penetrability through the skin. Hence, using nanoparticles in cosmetic productions improves the supplementation of skin, hair, or teeth with active cosmetic ingredients (APIs). It is important to note that utilizing nanoparticles for several applications, as an emerging field of science, causes various concerns about being toxic or harmful for the body or the environment. The cosmetic industry’s products are commonly designed for skin, hair, nail, teeth, and therefore, are directly related to the health of the human body. Thus, it is reasonable to assume that there are even more concerns about using nanoparticles in this industry compared to others (Santos et al. 2019 ).
In addition to these cases, nanotechnology can be useful for the detection of harmful components in cosmetic ingredients. Therefore the application of methods like covered iron oxide nanoparticles with silver for detection of mercury contamination in cosmetics (Chen et al. 2021a , b ), Quantitative assessment of the Triamcinolone acetonide (TCA) (which is a hazardous component in high doses) using nanoparticles with luminescence property (Zhang et al. 2019a ), And detection of harmful N-nitrosamines with the utilization of magnetic nanoparticles (Miralles et al. 2019 ) are worth mentioning.
Oil industry benefits from multiple types of nanomaterials
Nanomaterials can play a major role in the advancement of the oil industry. Almost every form of nanomaterial—discussed in previous sections—has been exhibited to have numerous applications in the oil industry. Nanomaterial can be effectively exploited in various processes of this industry, including oil exploration/production and recovering the oilfield. Nanofluids (synthesized from nanomaterials) optimize the oil production process. Nanocatalysts have applications in petrochemical processes along with operating an efficient oil purgation function. Several applications of this technology are mentioned below.
There are nanomembranes designed to provide a proper matrix for separating water and oil from gas. They eventually purify the gas and delete redundant components from wastewater (Saleh 2018 ). Metal workings such as machining and stamping industry require some types of lubricants and coolants, which are mostly oil products. There has been produced an oil-based cutting fluid made up of Al 2 O 3 nanoparticles to decrease the friction force between the object and snipping tool (Subhedar et al. 2021 ). Encapsulation of extracted essential oil from hyssop in a nano-complex improves the antioxidant and antifungal efficiency of the oil (Hadidi et al. 2021 ). The application of nano-silica in the procedure of oil cementing enhances the resistance of the cement (Goyal et al. 2021 ; Thakkar et al. 2020 ). In the process of oil recovery, there is a high energy loss that imposes damages to the injection system and lowers the heat level. To keep the rate of temperature in a higher range and decrease the energy loss, scientists have applied nano-thermal insulators that are more economical (Afra et al. 2021 ; Zhao et al. 2021 ; Zhou et al. 2020 ). Gas and oil products can be cleaned from H 2 S by applying nanomaterials (Agarwal and Sudharsan 2021 ). Utilizing starch nano coatings (Wang et al. 2021 ), Lignin and nano-silica (Gong et al. 2021 ), Lotus leaf coated with nano-SiO 2 (Yang et al. 2021 ), and nano zeolite membrane are new methods for the separation of oil and water due to their high hydrophobic property (Anis et al. 2021 ). Nanotechnology can be used to improve the quality of engine oil, which results in the better stability and lubricity power as well as a reduced rate of released carbon mono oxide (Tonk 2021 ; Saidi et al. 2021 ; Thirugnanam et al. 2021 ; Ardebili et al. 2020 ). Advanced nanoemulsions show high stability and benefits for the oil industry due to the larger surface and the ability to wet (Kumar et al. 2021 ). Encapsulation of essential oils in nanostructures indicates a better performance as a pesticide due to better maintenance of the oil (Campolo et al. 2020 ). Producing an oil-in-water emulsion by applying protein nanoparticles can protect unstable and active ingredients and benefit the medicine and food industry (Xu et al. 2020 ).
Combining diverse fields of science in a manner that they overcome each other’s deficiencies indicates promising results. Within the last decades, biotechnology has made a lot of progress. Merging nanotechnology with biotechnological methods enables scientists to design less time taking, more economical, and more efficient techniques. This Nano-biotechnological approach influences multiple therapeutic, agricultural, environmental, and industrial methods. For instance, the effectiveness of the emergent crisper/cas9 systems increases noticeably by applying the nano-scaled additives at the process.
In this review, we investigated the current advancements and limitations of biotechnology, along with the nano-based alternatives rendered by nanotechnology. It seems highly probable that biotechnology will accomplish even more improvements in the future, and its incorporation with nanotechnology gets humankind one step closer to a sustainable future. Besides, the nano-based techniques are less costly compared to the conventional ones. Thus, with nano-biotechnology promoting, a revolution in the economic situation of the world is not implausible.
Author contributions
All authors have participated in (a) conception and design, or analysis and interpretation of the data; (b) drafting the article or revising it critically for important intellectual content; and (c) approval of the final version. This manuscript has not been submitted to, nor is under review at, another journal or other publishing venue. The authors have no affiliation with any organization with a direct or indirect financial interest in the subject matter discussed in the manuscript.
Declarations
On behalf of all authors, the corresponding author states that there is no conflict of interest.
Contributor Information
Nikta Shahcheraghi, Email: [email protected] .
Hasti Golchin, Email: moc.liamg@87nihclogitsah .
Zahra Sadri, Email: moc.liamg@6707irdasarhaz .
Yasaman Tabari, Email: [email protected] .
Forough Borhanifar, Email: [email protected] .
Shadi Makani, Email: [email protected] .
- Abass OK, Zhang K. Nano-Fe mediated treatment of real hydraulic fracturing flowback and its practical implication on membrane fouling in tandem anaerobic-oxic membrane bioreactor. J Hazard Mater. 2020; 395 :122666. [ PubMed ] [ Google Scholar ]
- Abdelhamid HN, Badr G. Nanobiotechnology as a platform for the diagnosis of COVID-19: a review. Nanotechnol Environ Eng. 2021; 6 (1):1–26. [ Google Scholar ]
- Adam H, Youssef A (2019) Economic impacts of nanotechnology industry: case study on Egypt. In: Proceedings of 10th International Conference on Digital Strategies for Organizational Success. 10.2139/ssrn.3314931
- Adeel M, Bilal M, Rasheed T, Sharma A, Iqbal HMN. Graphene and graphene oxide: functionalization and nano-bio-catalytic system for enzyme immobilization and biotechnological perspective. Int J Biol Macromol. 2018; 120 (Pt B):1430–1440. [ PubMed ] [ Google Scholar ]
- Afra M, Peyghambarzadeh SM, Shahbazi K, Tahmassebi N. Thermo-economic optimization of steam injection operation in enhanced oil recovery (EOR) using nano-thermal insulation. Energy. 2021; 226 :120409. [ Google Scholar ]
- Agarwal M, Sudharsan J. A comprehensive review on scavenging H 2 S compounds in oil and gas industry by using nanomaterials. Mater Today Proc. 2021 doi: 10.1016/j.matpr.2020.11.693. [ CrossRef ] [ Google Scholar ]
- Ahire JJ, Dicks LMT. 2,3-dihydroxybenzoic acid-containing nanofiber wound dressings inhibit biofilm formation by Pseudomonas aeruginosa . Antimicrob Agents Chemother. 2014; 58 (4):2098–2104. [ PMC free article ] [ PubMed ] [ Google Scholar ]
- Ahire JJ, Dicks LM. Antimicrobial hyaluronic acid–cefoxitin sodium thin films produced by electrospraying. Curr Microbiol. 2016; 73 (2):236–241. [ PubMed ] [ Google Scholar ]
- Ahire JJ, Neveling DP. Dicks LM (2018) Polyacrylonitrile (PAN) nanofibres spun with copper nanoparticles: an anti- Escherichia coli membrane for water treatment. Appl Microbiol Biotechnol. 2018; 102 (16):7171–7181. [ PubMed ] [ Google Scholar ]
- Ahire JJ, Hattingh M, Neveling DP, Dicks LM. Copper-containing anti-biofilm nanofiber scaffolds as a wound dressing material. PLoS ONE. 2016; 11 (3):e0152755. [ PMC free article ] [ PubMed ] [ Google Scholar ]
- Ahire JJ, Robertson DD, van Reenen AJ, Dicks LMT. Polyethylene oxide (PEO)-hyaluronic acid (HA) nanofibers with kanamycin inhibits the growth of Listeria monocytogenes. Biomed Pharmacother. 2017; 86 :143–148. [ PubMed ] [ Google Scholar ]
- Ahire JJ, Robertson DD, Van Reenen AJ, Dicks LM. Surfactin-loaded polyvinyl alcohol (PVA) nanofibers alters adhesion of Listeria monocytogenes to polystyrene. Mater Sci Eng C. 2017; 77 :27–33. [ PubMed ] [ Google Scholar ]
- Ahmadi MH, Ghazvini M, Alhuyi Nazari M, Ahmadi MA, Pourfayaz F, Lorenzini G, Ming T. Renewable energy harvesting with the application of nanotechnology: a review. Int J Energy Res. 2019; 43 (4):1387–1410. [ Google Scholar ]
- Al-Attabi R, Rodriguez-Andres J, Schütz JA, Bechelany M, Des Ligneris E, Chen X, Kong L, Morsi YS, Dumee LF. Catalytic electrospun nano-composite membranes for virus capture and remediation. Sep Purif Technol. 2019; 229 :115806. [ Google Scholar ]
- Al-Gheethi AAS, Mohamed RMSR, Noman EA, Kassim AHM, editors. Prospects of fresh market wastes management in developing countries. Water science and technology library. Cham: Springer; 2020. [ Google Scholar ]
- Ali M, Hoque M, Safdar Hossain S, Biswas M. Nanoadsorbents for wastewater treatment: next generation biotechnological solution. Int J Environ Sci Technol. 2020; 17 :4095–4132. [ Google Scholar ]
- Ali SS, Al-Tohamy R, Koutra E, Moawad MS, Kornaros M, Mustafa AM, Mahmoud YA-G, Badr A, Osman ME, Elsamahy T. Nanobiotechnological advancements in agriculture and food industry: applications, nanotoxicity, and future perspectives. Sci Total Environ. 2021; 792 :148359. [ PubMed ] [ Google Scholar ]
- AlNadhari S, Al-Enazi NM, Alshehrei F, Ameen F. A review on biogenic synthesis of metal nanoparticles using marine algae and its applications. Environ Res. 2021; 194 :110672. [ PubMed ] [ Google Scholar ]
- Anis SF, Lalia BS, Lesimple A, Hashaikeh R, Hilal N. Superhydrophilic and underwater superoleophobic nano zeolite membranes for efficient oil-in-water nanoemulsion separation. J Water Process Eng. 2021; 40 :101802. [ Google Scholar ]
- Ardebili SMS, Taghipoor A, Solmaz H, Mostafaei M. The effect of nano-biochar on the performance and emissions of a diesel engine fueled with fusel oil-diesel fuel. Fuel. 2020; 268 :117356. [ Google Scholar ]
- Ashraf S, Siddiqa A, Shahida S, Qaisar S. Titanium-based nanocomposite materials for arsenic removal from water: a review. Heliyon. 2019; 5 (5):e01577. [ PMC free article ] [ PubMed ] [ Google Scholar ]
- Azarakhsh F, Divsalar A, Saboury AA, Eidi A (2021) Simultaneous Delivery of Oxali-palladium and Iron Nanoparticles by β-Casein. Journal of Molecular Liquids:115999
- Azeez NA, Dash SS, Gummadi SN, Deepa VS (2020) Nano-remediation of toxic heavy metal contamination: Hexavalent chromium [Cr (VI)]. Chemosphere:129204 [ PubMed ]
- Banerjee S, Dubey S, Gautam RK, Chattopadhyaya M, Sharma YC. Adsorption characteristics of alumina nanoparticles for the removal of hazardous dye, Orange G from aqueous solutions. Arab J Chem. 2019; 12 (8):5339–5354. [ Google Scholar ]
- Barani M, Bilal M, Sabir F, Rahdar A, Kyzas GZ. Nanotechnology in ovarian cancer: diagnosis and treatment. Life Sci. 2021; 266 :118914. [ PubMed ] [ Google Scholar ]
- Bashir F, Irfan M, Ahmad T, Iqbal J, Butt MT, Sadef Y, Umbreen M, Shaikh IA, Moniruzzaman M. Efficient utilization of low cost agro materials for incorporation of copper nanoparticles to scrutinize their antibacterial properties in drinking water. Environ Technol Innov. 2021; 21 :101228. [ Google Scholar ]
- Bayda S, Adeel M, Tuccinardi T, Cordani M, Rizzolio F. The history of nanoscience and nanotechnology: from chemical–physical applications to nanomedicine. Molecules. 2020; 25 (1):112. [ PMC free article ] [ PubMed ] [ Google Scholar ]
- Benrabah L, Kemel K, Twarog C, Huang N, Solgadi A, Laugel C, Faivre V. Lipid-based Janus nanoparticles for pharmaceutical and cosmetic applications: kinetics and mechanisms of destabilization with time and temperature. Colloids Surfaces B. 2020; 195 :111242. [ PubMed ] [ Google Scholar ]
- Bensaida K, Eljamal R, Sughihara Y, Eljamal O. The impact of iron bimetallic nanoparticles on bulk microbial growth in wastewater. J Water Process Eng. 2021; 40 :101825. [ Google Scholar ]
- Beryani A, Pardakhti A, Ardestani M, Zahed MA. Benzene and MTBE removal by Fenton’s process using stabilized Nano Zero-Valent Iron particles. J Appl Res Water Wastewater. 2017; 4 (2):343–348. doi: 10.22126/arww.2017.788. [ CrossRef ] [ Google Scholar ]
- Bhuyan D, Greene GW, Das RK. Prospects and application of nanobiotechnology in food preservation: molecular perspectives. Crit Rev Biotechnol. 2019; 39 (6):759–778. [ PubMed ] [ Google Scholar ]
- Biesiada M, Pachulska-Wieczorek K, Adamiak RW, Purzycka KJ. RNAComposer and RNA 3D structure prediction for nanotechnology. Methods. 2016; 103 :120–127. [ PubMed ] [ Google Scholar ]
- Borrás MC, Sluyter R, Barker PJ, Konstantinov K, Bakand S. Y2O3 decorated TiO2 nanoparticles: enhanced UV attenuation and suppressed photocatalytic activity with promise for cosmetic and sunscreen applications. J Photochem Photobiol B Biol. 2020; 207 :111883. [ PubMed ] [ Google Scholar ]
- Campolo O, Giunti G, Laigle M, Michel T, Palmeri V. Essential oil-based nano-emulsions: effect of different surfactants, sonication and plant species on physicochemical characteristics. Ind Crops Prod. 2020; 157 :112935. [ Google Scholar ]
- Cardoso VF, Francesko A, Ribeiro C, Bañobre-López M, Martins P, Lanceros-Mendez S. Advances in magnetic nanoparticles for biomedical applications. Adv Healthcare Mater. 2018; 7 (5):1700845. [ PubMed ] [ Google Scholar ]
- Chai JM, Amelia TSM, Mouriya GK, Bhubalan K, Amirul A-AA, Vigneswari S, Ramakrishna S. Surface-Modified highly biocompatible bacterial-poly (3-hydroxybutyrate-co-4-hydroxybutyrate): a review on the promising next-generation biomaterial. Polymers. 2021; 13 (1):51. [ PMC free article ] [ PubMed ] [ Google Scholar ]
- Chen H, Gu L, Liao B, Zhou X, Cheng L, Ren B. Advances of anti-caries nanomaterials. Molecules. 2020; 25 (21):5047. [ PMC free article ] [ PubMed ] [ Google Scholar ]
- Chen L, Ai J, Cai H, Chen X, Liu Z, Li Z, Dai F. Antibacterial gauze based on the synergistic antibacterial mechanism of antimicrobial peptides and silver nanoparticles. J Polym Res. 2021; 28 (2):1–9. [ Google Scholar ]
- Chen Z-Y, Gupta A, Chattopadhyay S. Detection of mercury in spiked cosmetics by surface enhanced Raman spectroscopy using silver shelled iron oxide nanoparticles. Sens Actuators B Chem. 2021; 337 :129788. [ Google Scholar ]
- Chhipa H. Applications of nanotechnology in agriculture. Methods Microbiol. 2019; 46 :115–142. [ Google Scholar ]
- Chiari-Andréo BG, Almeida-Cincotto MGJd, Oshiro JA, Taniguchi CYY, Chiavacci LA, Isaac VLB. Chapter 5—Nanoparticles for cosmetic use and its application. In: Grumezescu AM, editor. Nanoparticles in pharmacotherapy. William Andrew Publishing; 2019. pp. 113–146. [ Google Scholar ]
- Chugh G, Siddique KH, Solaiman ZM. Nanobiotechnology for agriculture: smart technology for combating nutrient deficiencies with nanotoxicity challenges. Sustainability. 2021; 13 (4):1781. [ Google Scholar ]
- Costa JSD, Hoskisson PA, Paterlini P, Romero CM, Alvarez A. Whole genome sequence of the multi-resistant plant growth-promoting bacteria Streptomyces sp. Z38 with potential application in agroindustry and bio-nanotechnology. Genomics. 2020; 112 (6):4684–4689. [ PubMed ] [ Google Scholar ]
- D’Acunto M, Cioni P, Gabellieri E, Presciuttini G. Exploiting gold nanoparticles for diagnosis and cancer treatments. Nanotechnology. 2021; 32 (19):192001. [ PubMed ] [ Google Scholar ]
- de Azevedo SA, Fonseca GO, Duarte PS, Macedo LC, Percebom AM. Nanoparticles of surfactant and block copolymers with high uptake of oily ingredients for cosmetic formulations. Colloids Surf A Physicochem Eng Asp. 2019; 581 :123779. [ Google Scholar ]
- De Luca I, Pedram P, Moeini A, Cerruti P, Peluso G, Di Salle A, Germann N. Nanotechnology development for formulating essential oils in wound dressing materials to promote the wound-healing process: a review. Appl Sci. 2021; 11 (4):1713. [ Google Scholar ]
- Debnath M, Chauhan N, Sharma P, Tomar I. Handbook of nanomaterials for wastewater treatment. Elsevier; 2021. Potential of nano biosurfactants as an ecofriendly green technology for bioremediation; pp. 1039–1055. [ Google Scholar ]
- Deng H, Huang W, Zhang Z. Nanotechnology based CRISPR/Cas9 system delivery for genome editing: progress and prospect. Nano Res. 2019; 12 (10):2437–2450. [ Google Scholar ]
- Desai AV, Sharma S, Ghosh SK. Metal-organic frameworks (MOFs) for environmental applications. Elsevier; 2019. Metal-organic frameworks for recognition and sequestration of toxic anionic pollutants; pp. 95–140. [ Google Scholar ]
- Ding S-s, He L, Bian X-w, Tian G. Metal-organic frameworks-based nanozymes for combined cancer therapy. Nano Today. 2020; 35 :100920. [ Google Scholar ]
- Doroudian M, MacLoughlin R, Poynton F, Prina-Mello A, Donnelly SC. Nanotechnology based therapeutics for lung disease. Thorax. 2019; 74 (10):965–976. [ PubMed ] [ Google Scholar ]
- Doroudian M, O’Neill A, Mac Loughlin R, Prina-Mello A, Volkov Y, Donnelly SC. Nanotechnology in pulmonary medicine. Curr Opin Pharmacol. 2021; 56 :85–92. [ PMC free article ] [ PubMed ] [ Google Scholar ]
- Du T, Zhang J, Li C, Song T, Li P, Liu J, Du X, Wang S. Gold/silver hybrid nanoparticles with enduring inhibition of coronavirus multiplication through multisite mechanisms. Bioconjug Chem. 2020; 31 (11):2553–2563. [ PubMed ] [ Google Scholar ]
- Ebrahimbabaie P, Meeinkuirt W, Pichtel J. Phytoremediation of engineered nanoparticles using aquatic plants: mechanisms and practical feasibility. J Environ Sci. 2020; 93 :151–163. [ PubMed ] [ Google Scholar ]
- Evelyn Roopngam P. Liposome and polymer-based nanomaterials for vaccine applications. Nanomed J. 2019; 6 (1):1–10. [ Google Scholar ]
- Farrera C, Torres Andón F, Feliu N. Carbon nanotubes as optical sensors in biomedicine. ACS Nano. 2017; 11 (11):10637–10643. [ PubMed ] [ Google Scholar ]
- Fidoski J, Benedetti A, Kirkov A, Iliev A, Stamatoski A, Baftijari D. Nano-emulsion complex (propolis and vitamin C) promotes wound healing in the oral mucosa. Oral Maxillofac Patho J. 2020; 11 (1):1–5. [ Google Scholar ]
- Fouad GI. A proposed insight into the anti-viral potential of metallic nanoparticles against novel coronavirus disease-19 (COVID-19) Bull Natl Res Cent. 2021; 45 (1):1–22. [ PMC free article ] [ PubMed ] [ Google Scholar ]
- Furtado D, Björnmalm M, Ayton S, Bush AI, Kempe K, Caruso F. Overcoming the blood–brain barrier: the role of nanomaterials in treating neurological diseases. Adv Mater. 2018; 30 (46):1801362. [ PubMed ] [ Google Scholar ]
- Gangadoo S, Nguyen H, Rajapaksha P, Zreiqat H, Latham K, Cozzolino D, Truong VK. Inorganic nanoparticles as food additives and their influence on the human gut microbiota. Environ Sci Nano. 2021; 8 :1500–1518. [ Google Scholar ]
- Ghebretatios M, Schaly S, Prakash S. Nanoparticles in the food industry and their impact on human gut microbiome and diseases. Int J Mol Sci. 2021; 22 (4):1942. [ PMC free article ] [ PubMed ] [ Google Scholar ]
- Gökçe D. Influences of nanoparticles on aquatic organisms: current situation of nanoparticles effects in aquatic ecosystems. Sust Eng Innov. 2021; 3 (1):54–60. [ Google Scholar ]
- Gong X, Meng Y, Zhu J, Wang X, Lu J, Cheng Y, Tao Y, Wang H. Construct a stable super-hydrophobic surface through acetonitrile extracted lignin and nano-silica and its application in oil-water separation. Ind Crops Prod. 2021; 166 :113471. [ Google Scholar ]
- González-Ballesteros N, Prado-López S, Rodríguez-González J, Lastra M, Rodríguez-Argüelles M. Green synthesis of gold nanoparticles using brown algae Cystoseira baccata : Its activity in colon cancer cells. Colloids Surf B. 2017; 153 :190–198. [ PubMed ] [ Google Scholar ]
- Goracci M, Pignochino Y, Marchiò S. Phage display-based nanotechnology applications in cancer immunotherapy. Molecules. 2020; 25 (4):843. [ PMC free article ] [ PubMed ] [ Google Scholar ]
- Goyal S, Joshi P, Singh R. Applications and role of Nano-Silica particles on altering the properties and their usage for oil well cementing. Mater Today Proc. 2021; 46 :10681–10686. [ Google Scholar ]
- Hadidi M, Motamedzadegan A, Jelyani AZ, Khashadeh S. Nanoencapsulation of hyssop essential oil in chitosan-pea protein isolate nano-complex. LWT. 2021; 144 :111254. [ Google Scholar ]
- Hager S, Fittler FJ, Wagner E, Bros M. Nucleic acid-based approaches for tumor therapy. Cells. 2020; 9 (9):2061. [ PMC free article ] [ PubMed ] [ Google Scholar ]
- Hajiabadi SH, Aghaei H, Kalateh-Aghamohammadi M, Shorgasthi M. An overview on the significance of carbon-based nanomaterials in upstream oil and gas industry. J Pet Sci Eng. 2020; 186 :106783. [ Google Scholar ]
- He Y, Lin H, Luo M, Liu J, Dong Y, Li B. Highly efficient remediation of groundwater co-contaminated with Cr (VI) and nitrate by using nano-Fe/Pd bimetal-loaded zeolite: process product and interaction mechanism. Environ Pollut. 2020; 263 :114479. [ PubMed ] [ Google Scholar ]
- Hosseini SF, Ramezanzade L, McClements DJ. Recent advances in nanoencapsulation of hydrophobic marine bioactives: bioavailability, safety, and sensory attributes of nano-fortified functional foods. Trends Food Sci Technol. 2021; 109 :322–339. [ Google Scholar ]
- Hou S, Wu B, Peng D, Wang Z, Wang Y, Xu H. Remediation performance and mechanism of hexavalent chromium in alkaline soil using multi-layer loaded nano-zero-valent iron. Environ Pollut. 2019; 252 :553–561. [ PubMed ] [ Google Scholar ]
- Islam MA, Rice J, Reesor E, Zope H, Tao W, Lim M, Ding J, Chen Y, Aduluso D, Zetter BR. Adjuvant-pulsed mRNA vaccine nanoparticle for immunoprophylactic and therapeutic tumor suppression in mice. Biomaterials. 2021; 266 :120431. [ PMC free article ] [ PubMed ] [ Google Scholar ]
- Iwaniec DM, Cook EM, Davidson MJ, Berbés-Blázquez M, Georgescu M, Krayenhoff ES, Middel A, Sampson DA, Grimm NB. The co-production of sustainable future scenarios. Landsc Urban Plan. 2020; 197 :103744. [ Google Scholar ]
- Jafari SM, Arpagaus C, Cerqueira MA, Samborska K. Nano spray drying of food ingredients; materials, processing and applications. Trends Food Sci Technol. 2021; 109 :632–646. [ Google Scholar ]
- Jang W, Kwon H, Park Y, Lee H. Predicting the degree of interdisciplinarity in academic fields: the case of nanotechnology. Scientometrics. 2018; 116 (1):231–254. [ Google Scholar ]
- Jeevanandam J, Pal K, Danquah MK. Virus-like nanoparticles as a novel delivery tool in gene therapy. Biochimie. 2019; 157 :38–47. [ PubMed ] [ Google Scholar ]
- Jeong YJ, Kim DS, Kim JY, Oyunbaatar NE, Shanmugasundaram A, Kim ES, Lee DW. On-stage bioreactor platform integrated with nano-patterned and gold-coated PDMS diaphragm for live cell stimulation and imaging. Mater Sci Eng C-Mater Biol Appl. 2021; 118 :9. doi: 10.1016/j.msec.2020.111355. [ PubMed ] [ CrossRef ] [ Google Scholar ]
- Jiang Y, Khan A, Huang H, Tian Y, Yu X, Xu Q, Mou L, Lv J, Zhang P, Liu P, Deng L, Li X. Using nano-attapulgite clay compounded hydrophilic urethane foams (AT/HUFs) as biofilm support enhances oil-refinery wastewater treatment in a biofilm membrane bioreactor. Sci Total Environ. 2019; 646 :606–617. [ PubMed ] [ Google Scholar ]
- Jogee P, Agarkar G, Rai M. Biopolymer-based nano films. Elsevier; 2021. Starch-based films loaded with nano-antimicrobials for food packaging; pp. 99–114. [ Google Scholar ]
- Jun B-M, Al-Hamadani YA, Son A, Park CM, Jang M, Jang A, Kim NC, Yoon Y. Applications of metal-organic framework based membranes in water purification: a review. Sep Purif Technol. 2020; 247 :116947. [ Google Scholar ]
- Kailasa SK, Mehta VN, Koduru JR, Basu H, Singhal RK, Murthy Z, Park T-J. An overview of molecular biology and nanotechnology based analytical methods for the detection of SARS-CoV-2: promising biotools for the rapid diagnosis of COVID-19. Analyst. 2021; 146 (5):1489–1513. [ PubMed ] [ Google Scholar ]
- Karimzadeh Z, Javanbakht S, Namazi H. Carboxymethylcellulose/MOF-5/Graphene oxide bio-nanocomposite as antibacterial drug nanocarrier agent. BioImpacts. 2019; 9 (1):5. [ PMC free article ] [ PubMed ] [ Google Scholar ]
- Ken DS, Sinha A. Recent developments in surface modification of nano zero-valent iron (nZVI): remediation, toxicity and environmental impacts. Environ Nanotechnol Monit Manag. 2020; 24 :100344. [ Google Scholar ]
- Khezri K, Saeedi M, Dizaj SM. Application of nanoparticles in percutaneous delivery of active ingredients in cosmetic preparations. Biomed Pharmacother. 2018; 106 :1499–1505. [ PubMed ] [ Google Scholar ]
- Kim S, Kim K, Jun G, Hwang W. Wood-nanotechnology-based membrane for the efficient purification of oil-in-water emulsions. ACS Nano. 2020; 14 (12):17233–17240. [ PubMed ] [ Google Scholar ]
- Kinik FP, Kampouri S, Ebrahim FM, Valizadeh B, Stylianou KC. Porous metal-organic frameworks for advanced applications. Elsevier; 2020. [ Google Scholar ]
- Kinloch IA, Suhr J, Lou J, Young RJ, Ajayan PM. Composites with carbon nanotubes and graphene: an outlook. Science. 2018; 362 (6414):547–553. [ PubMed ] [ Google Scholar ]
- Korde JM, Kandasubramanian B. Microbiologically extracted poly (hydroxyalkanoates) and its amalgams as therapeutic nano-carriers in anti-tumor therapies. Mater Sci Eng C. 2020; 111 :110799. [ PubMed ] [ Google Scholar ]
- Kumar S, Kashyap PL, Mahapatra S, Jasrotia P, Singh GP. New and emerging technologies for detecting Magnaporthe oryzae causing blast disease in crop plants. Crop Prot. 2020; 143 :105473. [ Google Scholar ]
- Kumar N, Verma A, Mandal A. Formation, characteristics and oil industry applications of nanoemulsions: a review. J Pet Sci Eng. 2021; 206 :109042. [ Google Scholar ]
- Kutara BMRNS, Sutharb H, Mohitec KC, Devid R (2016) Bioinformatics, biotechnology and nanotechnology tools for environment engineering: way to sustainable development by wastewater treatment
- Landowski LM, Niego B, Sutherland BA, Hagemeyer CE, Howells DW. Applications of nanotechnology in the diagnosis and therapy of stroke. Semin Thromb Hemost. 2020; 46 (5):592–605. doi: 10.1055/s-0039-3399568. [ PubMed ] [ CrossRef ] [ Google Scholar ]
- Le TT, Yoon H, Son M-H, Kang Y-G, Chang Y-S. Treatability of hexabromocyclododecane using Pd/Fe nanoparticles in the soil-plant system: effects of humic acids. Sci Total Environ. 2019; 689 :444–450. [ PubMed ] [ Google Scholar ]
- Lew TTS, Koman VB, Gordiichuk P, Park M, Strano MS. The emergence of plant nanobionics and living plants as technology. Adv Mater Technol. 2020; 5 (3):1900657. [ Google Scholar ]
- Li T, Fang F, Yang Y, Shen W, Bao W, Zhang T, Ai F, Ding X, Xin H, Wang X. Surface nano-engineered wheat straw for portable and adjustable water purification. Sci Total Environ. 2019; 655 :1028–1036. [ PubMed ] [ Google Scholar ]
- Li R, Ng TS, Wang SJ, Prytyskach M, Rodell CB, Mikula H, Kohler RH, Garlin MA, Lauffenburger DA, Parangi S. Therapeutically reprogrammed nutrient signalling enhances nanoparticulate albumin bound drug uptake and efficacy in KRAS-mutant cancer. Nat Nanotechnol. 2021 doi: 10.1038/s41565-021-00897-1. [ PMC free article ] [ PubMed ] [ CrossRef ] [ Google Scholar ]
- Lian M, Wang L, Feng Q, Niu L, Zhao Z, Wang P, Song C, Li X, Zhang Z. Thiol-functionalized nano-silica for in-situ remediation of Pb, Cd, Cu contaminated soils and improving soil environment. Environ Pollut. 2021; 280 :116879. [ PubMed ] [ Google Scholar ]
- Lim M, Badruddoza AZM, Firdous J, Azad M, Mannan A, Al-Hilal TA, Cho C-S, Islam MA. Engineered nanodelivery systems to improve DNA vaccine technologies. Pharmaceutics. 2020; 12 (1):30. [ PMC free article ] [ PubMed ] [ Google Scholar ]
- Lin Y-X, Wang Y, Blake S, Yu M, Mei L, Wang H, Shi J. RNA nanotechnology-mediated cancer immunotherapy. Theranostics. 2020; 10 (1):281–299. doi: 10.7150/thno.35568. [ PMC free article ] [ PubMed ] [ CrossRef ] [ Google Scholar ]
- Lincy A, Jegathambal P, Mkandawire M, MacQuarrie S. Nano bioremediation of textile dye effluent using magnetite nanoparticles encapsulated alginate beads. J Environ Treat Tech. 2020; 8 (3):936–946. [ Google Scholar ]
- Liu B, Hu F, Zhang JF, Wang CL, Li LL. A biomimetic coordination nanoplatform for controlled encapsulation and delivery of drug-gene combinations. Angew Chem-Int Edit. 2019; 58 (26):8804–8808. [ PubMed ] [ Google Scholar ]
- Lohrasebi A, Koslowski T. Modeling water purification by an aquaporin-inspired graphene-based nano-channel. J Mol Model. 2019; 25 (9):280. [ PubMed ] [ Google Scholar ]
- Lombardo D, Kiselev MA, Caccamo MT. Smart nanoparticles for drug delivery application: development of versatile nanocarrier platforms in biotechnology and nanomedicine. J Nanomater. 2019 doi: 10.1155/2019/3702518. [ CrossRef ] [ Google Scholar ]
- Luo Y, Wang Q, Zhang Y. Biopolymer-based nanotechnology approaches to deliver bioactive compounds for food applications: a perspective on the past, present, and future. J Agric Food Chem. 2020; 68 (46):12993–13000. [ PubMed ] [ Google Scholar ]
- Luo Z, Zhu J, Yu L, Yin K. Heavy metal remediation by nano zero-valent iron in the presence of microplastics in groundwater: inhibition and induced promotion on aging effects. Environ Pollut. 2021; 287 :117628. [ PubMed ] [ Google Scholar ]
- Lyu P, Javidi-Parsijani P, Atala A, Lu B. Delivering Cas9/sgRNA ribonucleoprotein (RNP) by lentiviral capsid-based bionanoparticles for efficient ‘hit-and-run’genome editing. Nucleic Acids Res. 2019; 47 (17):e99–e99. [ PMC free article ] [ PubMed ] [ Google Scholar ]
- Ma J, Dai R, Chen M, Khan SJ, Wang Z. Applications of membrane bioreactors for water reclamation: micropollutant removal, mechanisms and perspectives. Biores Technol. 2018; 269 :532–543. [ PubMed ] [ Google Scholar ]
- Mahmoud ME, Allam EA, El-Sharkawy RM, Soliman MA, Saad EA, El-Khatib AM. Nano-manganese oxide-functionalized-oleyl amine as a simple and low cost nanosorbent for remediation of ZnII/CoII and their radioactive nuclides 65Zn and 60Co from water. Appl Radiat Isot. 2020; 159 :108989. [ PubMed ] [ Google Scholar ]
- Maine E, Thomas V, Bliemel M, Murira A, Utterback J. The emergence of the nanobiotechnology industry. Nat Nanotechnol. 2014; 9 (1):2–5. [ PubMed ] [ Google Scholar ]
- Majhi RK, Mohanty S, Khan MI, Mishra A, Brauner A. Ag@ ZnO nanoparticles induce antimicrobial peptides and promote migration and antibacterial activity of keratinocytes. ACS Infect Dis. 2021 doi: 10.1021/acsinfecdis.0c00903. [ PMC free article ] [ PubMed ] [ CrossRef ] [ Google Scholar ]
- Malik SN, Khan SM, Ghosh PC, Vaidya AN, Kanade G, Mudliar SN. Treatment of pharmaceutical industrial wastewater by nano-catalyzed ozonation in a semi-batch reactor for improved biodegradability. Sci Total Environ. 2019; 678 :114–122. [ PubMed ] [ Google Scholar ]
- Manikanika KJ, Jaswal S. Role of nanotechnology in the world of cosmetology: a review. Mater Today Proc. 2021 doi: 10.1016/j.matpr.2020.12.638. [ CrossRef ] [ Google Scholar ]
- Mantatov V, Mantatova L, Nasibulina A, Tutubalin V. The conception of sustainable development in the light of converging technologies. Eur Asian J Bio Sci. 2019; 13 (2):2159–2165. [ Google Scholar ]
- Marchiol L. Nanotechnology in agriculture: new opportunities and perspectives. New Visions Plant Sci. 2018; 9 (4):161. [ Google Scholar ]
- Marcos-Contreras OA, Greineder CF, Kiseleva RY, Parhiz H, Walsh LR, Zuluaga-Ramirez V, Myerson JW, Hood ED, Villa CH, Tombacz I, Pardi N, Seliga A, Mui BL, Tam YK, Glassman PM, Shuvaev VV, Nong J, Brenner JS, Khoshnejad M, Madden T, Weissmann D, Persidsky Y, Muzykantov VR. Selective targeting of nanomedicine to inflamed cerebral vasculature to enhance the blood–brain barrier. Proc Natl Acad Sci. 2020; 117 (7):3405–3414. [ PMC free article ] [ PubMed ] [ Google Scholar ]
- Meikle TG, Dharmadana D, Hoffmann SV, Jones NC, Drummond CJ, Conn CE. Analysis of the structure, loading and activity of six antimicrobial peptides encapsulated in cubic phase lipid nanoparticles. J Colloid Interface Sci. 2021; 587 :90–100. [ PubMed ] [ Google Scholar ]
- Milinčić DD, Popović DA, Lević SM, Kostić A, Tešić ŽL, Nedović VA, Pešić MB. Application of polyphenol-loaded nanoparticles in food industry. Nanomaterials. 2019 doi: 10.3390/nano9111629. [ PMC free article ] [ PubMed ] [ CrossRef ] [ Google Scholar ]
- Miralles P, van Gemert I, Chisvert A, Salvador A. Stir bar sorptive-dispersive microextraction mediated by magnetic nanoparticles-metal organic framework composite: determination of N-nitrosamines in cosmetic products. J Chromatogr A. 2019; 1604 :460465. [ PubMed ] [ Google Scholar ]
- Mishra R, Manral A. Graphene based biopolymer nanocomposites. Springer; 2021. Graphene functionalized starch biopolymer nanocomposites: fabrication, characterization, and applications; pp. 173–189. [ Google Scholar ]
- Mishra A, Kumar J, Melo JS, Sandaka BP. Progressive development in biosensors for detection of dichlorvos pesticide: a review. J Environ Chem Eng. 2021; 9 (2):105067. [ Google Scholar ]
- Mofijur M, Siddiki SYA, Ahmed MB, Djavanroodi F, Fattah IR, Ong HC, Chowdhury M, Mahlia T. Effect of nanocatalysts on the transesterification reaction of first, second and third generation biodiesel sources-A mini-review. Chemosphere. 2020; 270 :128642. [ PubMed ] [ Google Scholar ]
- Mohid SA, Bhunia A. Combining antimicrobial peptides with nanotechnology: an emerging field in theranostics. Curr Protein Pept Sci. 2021; 21 (4):413–428. [ PubMed ] [ Google Scholar ]
- Murshid S, Gopinath KP, Prakash DG. Current nanotechnology based solutions for sustainable wastewater treatment. Curr Anal Chem. 2021; 17 (2):166–184. [ Google Scholar ]
- Nasrollahzadeh M, Sajjadi M, Iravani S, Varma RS. Green-synthesized nanocatalysts and nanomaterials for water treatment: current challenges and future perspectives. J Hazard Mater. 2020; 401 :123401. [ PMC free article ] [ PubMed ] [ Google Scholar ]
- Nawaz MZ, Attique SA, Alshammari FA, Alhamdi HW, Alghamdi HA, Yan W. A nanoinformatics approach to evaluate the pharmacological properties of nanoparticles for the treatment of Alzheimer's disease. Comb Chem High Throughput Screening. 2021 doi: 10.2174/1386207324666210217145733. [ PubMed ] [ CrossRef ] [ Google Scholar ]
- Ndlovu N, Mayaya T, Muitire C, Munyengwa N. Nanotechnology applications in crop production and food systems. Int J Plant Breeding Crop Sci. 2020; 7 (1):624–634. [ Google Scholar ]
- Nehra M, Dilbaghi N, Marrazza G, Kaushik A, Sonne C, Kim K-H, Kumar S. Emerging nanobiotechnology in agriculture for the management of pesticide residues. J Hazard Mater. 2021; 401 :123369. [ PubMed ] [ Google Scholar ]
- Nguyen VT, Vu QH, Pham TN, Trinh KS. Antibacterial filtration using polyethylene terephthalate filters coated with copper nanoparticles. J Nanomater. 2021 doi: 10.1155/2021/6628362. [ CrossRef ] [ Google Scholar ]
- Nizami A-S, Rehan M. Towards nanotechnology-based biofuel industry. Biofuel Res J. 2018; 5 (2):798. [ Google Scholar ]
- Oliveira AG, de Lara AJ, Montanha MC, Ogawa CYL, de Souza Freitas TKF, Moraes JCG, Sato F, Lima SM, da Cunha Andrade LH, Hechenleitner AAW. Wastewater treatment using Mg-doped ZnO nano-semiconductors: a study of their potential use in environmental remediation. J PhotochemPhotobiol A Chem. 2021; 407 :113078. [ Google Scholar ]
- Ozogul F, Elabed N, Ceylan Z, Ocak E, Ozogul Y. Nano-technological approaches for plant and marine-based polysaccharides for nano-encapsulations and their applications in food industry. Elsevier; 2021. pp. 187–236. [ PubMed ] [ Google Scholar ]
- Paiva-Santos AC, Herdade AM, Guerra C, Peixoto D, Pereira-Silva M, Zeinali M, Mascarenhas-Melo F, Paranhos A, Veiga F. Plant-mediated green synthesis of metal-based nanoparticles for dermopharmaceutical and cosmetic applications. Int J Pharm. 2021; 597 :120311. [ PubMed ] [ Google Scholar ]
- Patel RM, Dahane TM, Godbole S, Kambala SS, Mangal K. Applications of nanotechnology in prosthodontics. J Evol Med Dent Sci. 2020; 9 (47):3566–3572. [ Google Scholar ]
- Patra Shahi M, Kumari P, Mahobiya D, Kumar Shahi S. Chapter 4—nano-bioremediation of environmental contaminants: applications, challenges, and future prospects. In: Kumar V, Saxena G, Shah MP, editors. Bioremediation for environmental sustainability. Elsevier; 2021. pp. 83–98. [ Google Scholar ]
- Paunovska K, Loughrey D, Sago CD, Langer R, Dahlman JE. Using large datasets to understand nanotechnology. Adv Mater. 2019; 31 (43):1902798. [ PMC free article ] [ PubMed ] [ Google Scholar ]
- Peer D, Karp JM, Hong S, Farokhzad OC, Margalit R, Langer R. Nano-enabled medical applications. Jenny Stanford Publishing; 2020. Nanocarriers as an emerging platform for cancer therapy; pp. 61–91. [ PubMed ] [ Google Scholar ]
- Rabiee N, Bagherzadeh M, Ghasemi A, Zare H, Ahmadi S, Fatahi Y, Dinarvand R, Rabiee M, Ramakrishna S, Shokouhimehr M. Point-of-use rapid detection of sars-cov-2: nanotechnology-enabled solutions for the COVID-19 pandemic. Int J Mol Sci. 2020; 21 (14):5126. [ PMC free article ] [ PubMed ] [ Google Scholar ]
- Raghav S, Yadav PK, Kumar D. Handbook of nanomaterials for manufacturing applications. Elsevier; 2020. Nanotechnology for a sustainable future; pp. 465–492. [ Google Scholar ]
- Razavi R, Kenari RE, Farmani J, Jahanshahi M. Fabrication of zein/alginate delivery system for nanofood model based on pumpkin. Int J Biol Macromol. 2020; 165 (Pt B):3123–3134. [ PubMed ] [ Google Scholar ]
- Reshmy R, Philip E, Madhavan A, Sindhu R, Pugazhendhi A, Binod P, Sirohi R, Awasthi MK, Tarafdar A, Pandey A. Advanced biomaterials for sustainable applications in the food industry: updates and challenges. Environ Pollut. 2021; 283 :117071. [ PubMed ] [ Google Scholar ]
- Rezaei M, Shokuhfar A, Shahcheraghi N. Defect and diffusion forum. Trans Tech Publications; 2021. Preparation and photocatalytic performance of flower-shaped Zno/GO/Fe 3 O 4 on degradation of rhodamine B; pp. 161–172. [ Google Scholar ]
- Roco MC, Bainbridge WS. Converging technologies for improving human performance: nanotechnology, biotechnology, information technology and cognitive science. Springer Science Business Media; 2013. [ Google Scholar ]
- Rottenberg S, Disler C, Perego P. The rediscovery of platinum-based cancer therapy. Nat Rev Cancer. 2021; 21 (1):37–50. [ PubMed ] [ Google Scholar ]
- Rovera C, Ghaani M, Farris S. Nano-inspired oxygen barrier coatings for food packaging applications: an overview. Trends Food Sci Technol. 2020; 97 :210–220. [ Google Scholar ]
- Ruan X, Liu D, Niu X, Wang Y, Simpson CD, Cheng N, Du D, Lin Y. 2D Graphene oxide/Fe-MOF nanozyme nest with superior peroxidase-like activity and its application for detection of woodsmoke exposure biomarker. Anal Chem. 2019; 91 (21):13847–13854. [ PubMed ] [ Google Scholar ]
- Saari NHM, Chua LS. Nanoengineering in the beverage industry. Elsevier; 2020. Nano-based products in beverage industry; pp. 405–436. [ Google Scholar ]
- Sahani S, Sharma YC. Advancements in applications of nanotechnology in global food industry. Food Chem. 2020; 342 :128318. [ PubMed ] [ Google Scholar ]
- Sahu J, Karri RR, Zabed HM, Shams S, Qi X. Current perspectives and future prospects of nano-biotechnology in wastewater treatment. Sep Purif Rev. 2021; 50 (2):139–158. [ Google Scholar ]
- Saidi MZ, El Moujahid C, Pasc A, Canilho N, Delgado-Sanchez C, Celzard A, Fierro V, Kouitat-Njiwa R, Chafik T. Enhanced tribological properties of wind turbine engine oil formulated with flower-shaped MoS2 nano-additives. Colloids Surfaces A. 2021; 620 :126509. [ Google Scholar ]
- Sajjadi M, Ahmadpoor F, Nasrollahzadeh M, Ghafuri H. Lignin-derived (nano) materials for environmental pollution remediation: current challenges and future perspectives. Int J Biol Macromol. 2021; 178 :394–423. [ PubMed ] [ Google Scholar ]
- Sakulwech S, Lourith N, Ruktanonchai U, Kanlayavattanakul M. Preparation and characterization of nanoparticles from quaternized cyclodextrin-grafted chitosan associated with hyaluronic acid for cosmetics. Asian J Pharm Sci. 2018; 13 (5):498–504. [ PMC free article ] [ PubMed ] [ Google Scholar ]
- Saleh TA. Nanotechnology in oil and gas industries. Cham: Springer; 2018. [ Google Scholar ]
- Saleh TA. Nanomaterials: classification, properties, and environmental toxicities. Environ Technol Innov. 2020; 20 :101067. [ Google Scholar ]
- Samrot AV, Sahithya CS, Selvarani J, Purayil SK, Ponnaiah P. A review on synthesis, characterization and potential biological applications of superparamagnetic iron oxide nanoparticles. Curr Res Green Sust Chem. 2020; 4 :100042. [ Google Scholar ]
- Sánchez-Rubio F, Soria-Meneses PJ, Jurado-Campos A, Bartolome-Garcia J, Gomez-Rubio V, Soler AJ, Arroyo-Jimenez M, Santander-Ortega M, Plaza-Oliver M, Lozano M. Nanotechnology in reproduction: vitamin E nanoemulsions for reducing oxidative stress in sperm cells. Free Radical Biol Med. 2020; 160 :47–56. [ PubMed ] [ Google Scholar ]
- Santos AC, Morais F, Simões A, Pereira I, Sequeira JAD, Pereira-Silva M, Veiga F, Ribeiro A. Nanotechnology for the development of new cosmetic formulations. Expert Opin Drug Deliv. 2019; 16 (4):313–330. [ PubMed ] [ Google Scholar ]
- Séby F. Metal and metal oxide nanoparticles in cosmetics and skin care products. Elsevier; 2021. [ Google Scholar ]
- Selivanovitch E, Douglas T. Virus capsid assembly across different length scales inspire the development of virus-based biomaterials. Curr Opin Virol. 2019; 36 :38–46. [ PMC free article ] [ PubMed ] [ Google Scholar ]
- Sharma A, Singh G, Arya SK. Biofuel cell nanodevices. Int J Hydrogen Energy. 2021; 46 (4):3270–3288. [ Google Scholar ]
- Singh R, Misra V, Mudiam MKR, Chauhan LKS, Singh RP. Degradation of γ-HCH spiked soil using stabilized Pd/Fe0 bimetallic nanoparticles: pathways, kinetics and effect of reaction conditions. J Hazard Mater. 2012; 237–238 :355–364. [ PubMed ] [ Google Scholar ]
- Subhedar DG, Patel YS, Ramani BM, Patange GS. An experimental investigation on the effect of Al 2 O 3 /cutting oil based nano coolant for minimum quantity lubrication drilling of SS 304. Cleaner Eng Technol. 2021; 3 :100104. [ Google Scholar ]
- Ta Q, Ting J, Harwood S, Browning N, Simm A, Ross K, Olier I, Al-Kassas R. Chitosan nanoparticles for enhancing drugs and cosmetic components penetration through the skin. Eur J Pharm Sci. 2021; 160 :105765. [ PubMed ] [ Google Scholar ]
- Tan H, Wang C, Li H, Peng D, Zeng C, Xu H. Remediation of hexavalent chromium contaminated soil by nano-FeS coated humic acid complex in combination with Cr-resistant microflora. Chemosphere. 2020; 242 :125251. [ PubMed ] [ Google Scholar ]
- Tang C-H. Assembly of food proteins for nano-encapsulation and delivery of nutraceuticals (a mini-review) Food Hydrocoll. 2021; 117 :106710. [ Google Scholar ]
- Tavakoli N, Divsalar A, Haertlé T, Sawyer L, Saboury AA, Muronetz V. Milk protein-based nanodelivery systems for the cancer treatment. J Nanost Chem. 2021; 11 :483–500. [ Google Scholar ]
- Thakkar A, Raval A, Chandra S, Shah M, Sircar A. A comprehensive review of the application of nano-silica in oil well cementing. Petroleum. 2020; 6 (2):123–129. [ Google Scholar ]
- Thangadurai D, Sangeetha J, Prasad R. Nanotechnology for food, agriculture, and environment. Springer International Publishing; 2020. [ Google Scholar ]
- Thirugnanam C, Dharmaprabhakaran T, Kalaimurugan K, Somasundaram D, Kumar AV, Ali MMJ. Emission analysis of Palmyrah seed oil bio-diesel with NiO nano-particles on CI engine. Mater Today Proc. 2021 doi: 10.1016/j.matpr.2021.01.87. [ CrossRef ] [ Google Scholar ]
- Thomas S, Kumar Mishra P, Talegaonkar S. Ceramic nanoparticles: fabrication methods and applications in drug delivery. Curr Pharm Des. 2015; 21 (42):6165–6188. [ PubMed ] [ Google Scholar ]
- Tiwari K, Singh R, Negi P, Dani R, Rawat A. Application of nanomaterials in food packaging industry: a review. Mater Today Proc. 2021 doi: 10.1016/j.matpr.2021.01.385. [ CrossRef ] [ Google Scholar ]
- Tonk R. The science and technology of using nano-materials in engine oil as a lubricant additives. Mater Today Proc. 2021; 37 :3475–3479. [ Google Scholar ]
- Usman M, Farooq M, Wakeel A, Nawaz A, Cheema SA, Ur Rehman H, Ashraf I, Sanaullah M. Nanotechnology in agriculture: current status, challenges and future opportunities. Sci Total Environ. 2020; 721 :137778. [ PubMed ] [ Google Scholar ]
- Valencia PM, Farokhzad OC, Karnik R, Langer R. Nano-enabled medical applications. Jenny Stanford Publishing; 2020. Microfluidic technologies for accelerating the clinical translation of nanoparticles; pp. 93–112. [ PMC free article ] [ PubMed ] [ Google Scholar ]
- Vázquez-Núñez E, Molina-Guerrero CE, Peña-Castro JM, Fernández-Luqueño F, de la Rosa-Álvarez M. Use of nanotechnology for the bioremediation of contaminants: a review. Processes. 2020; 8 (7):826. [ Google Scholar ]
- Visentin C, da Silva Trentin AW, Braun AB, Thomé A. Nano scale zero valent iron production methods applied to contaminated sites remediation: an overview of production and environmental aspects. J Hazard Mater. 2020; 410 :124614. [ PubMed ] [ Google Scholar ]
- Wang D, Chen Y. Critical review of the influences of nanoparticles on biological wastewater treatment and sludge digestion. Crit Rev Biotechnol. 2016; 36 (5):816–828. [ PubMed ] [ Google Scholar ]
- Wang Y, Zhang G. The preparation of modified nano-starch and its application in food industry. Food Res Int. 2020; 140 :110009. [ PubMed ] [ Google Scholar ]
- Wang S, Chen Y, Wang S, Li P, Mirkin CA, Farha OK. DNA-functionalized metal–organic framework nanoparticles for intracellular delivery of proteins. J Am Chem Soc. 2019; 141 (6):2215–2219. [ PMC free article ] [ PubMed ] [ Google Scholar ]
- Wang Y, Chen Y, Hu Y, Fang X. Site-specific covalent labeling of large RNAs with nanoparticles empowered by expanded genetic alphabet transcription. Proc Natl Acad Sci. 2020; 117 (37):22823–22832. [ PMC free article ] [ PubMed ] [ Google Scholar ]
- Wang F, Chang R, Ma R, Tian Y. Eco-friendly and superhydrophobic nano-starch based coatings for self-cleaning application and oil-water separation. Carbohydr Polym. 2021; 271 :118410. [ PubMed ] [ Google Scholar ]
- Weijie M, Chongnv W, Xuming P, Weixin J, Yuhang W, Benhui S. TiO2 nanoparticles and multi-walled carbon nanotubes monitoring and bioremediation potential using ciliates Pseudocohnilembus persalinus . Ecotoxicol Environ Saf. 2020; 187 :109825. [ PubMed ] [ Google Scholar ]
- Xu B, Liu C, Sun H, Wang X, Huang F. Oil-in-water Pickering emulsions using a protein nano-ring as high-grade emulsifiers. Colloids Surfaces B. 2020; 187 :110646. [ PubMed ] [ Google Scholar ]
- Yadwade R, Gharpure S, Ankamwar B. Nanotechnology in cosmetics pros and cons. Nano Express. 2021; 2 (2):022003. [ Google Scholar ]
- Yang Y, Li B, Peng J, Lei Z, Zhu E, Zhang X, Feng X, Mi Y. The demulsification of crude oil emulsion driven by a natural lotus leaf grafted with nano-SiO2. J Environ Chem Eng. 2021; 9 (4):105586. [ Google Scholar ]
- Yao L, Ouyang L, Lv J, Dai P, Zhu L. Rapid and sensitive SERS detection of food contaminants by using nano-Ag aggregates with controllable hydrophobicity. Microchem J. 2021; 166 :106221. [ Google Scholar ]
- Yue T, Liu J, Yang Y, Chen S. Feasibility of cleaning As-and U-containing soil of a gold mine by using biologically nano-sized FeS: implications for soil remediation technology. Environ Technol Innov. 2021 doi: 10.1016/j.eti.2021.101775. [ CrossRef ] [ Google Scholar ]
- Zahed MA, Revayati M, Shahcheraghi N, Maghsoudi F, Tabari Y. Modeling and optimization of biodiesel synthesis using TiO2–ZnO nanocatalyst and characteristics of biodiesel made from waste sunflower oil. Curr Res Green Sustain Chem. 2021; 4 :100223. [ Google Scholar ]
- Zahed MA, Movahed E, Khodayari A, Zanganeh S, Badamaki M. Biotechnology for carbon capture and fixation: critical review and future directions. J Environ Manag. 2021; 293 :112830. [ PubMed ] [ Google Scholar ]
- Zhang S, Yao T, Wang S, Feng R, Chen L, Zhu V, Hu G, Zhang H, Yang G. Upconversion luminescence nanoparticles-based immunochromatographic assay for quantitative detection of triamcinolone acetonide in cosmetics. Spectrochim Acta Part A Mol Biomol Spectrosc. 2019; 214 :302–308. [ PubMed ] [ Google Scholar ]
- Zhang X, Wu D, Zhou X, Yu Y, Liu J, Hu N, Wang H, Li G, Wu Y. Recent progress in the construction of nanozyme-based biosensors and their applications to food safety assay. TrAC Trends Analyt Chem. 2019; 121 :115668. [ Google Scholar ]
- Zhang SS, Bilal M, Zdarta J, Cui JD, Kumar A, Franco M, Ferreira LFR, Iqbal HMN. Biopolymers and nanostructured materials to develop pectinases-based immobilized nano-biocatalytic systems for biotechnological applications. Food Res Int. 2021; 140 :17. [ PubMed ] [ Google Scholar ]
- Zhao R, Heng M, Chen C, Li T, Shi Y, Wang J. Catalytic effects of Al2O3 nano-particles on thermal cracking of heavy oil during in-situ combustion process. J Pet Sci Eng. 2021 doi: 10.1016/j.petrol.2021.108978. [ CrossRef ] [ Google Scholar ]
- Zhironkin S, Demchenko S, Kayachev G, Taran E, Zhironkina O (2019) Convergent and nature-like technologies as the basis for sustainable development in the 21st Century. In: E3S Web of Conferences. EDP Sciences, p 03008
- Zhou M, Hou T, Li J, Yu S, Xu Z, Yin M, Wang J, Wang X. Self-propelled and targeted drug delivery of poly(aspartic acid)/Iron–zinc microrocket in the stomach. ACS Nano. 2019; 13 (2):1324–1332. [ PubMed ] [ Google Scholar ]
- Zhou Y, Wu X, Zhong X, Reagen S, Zhang S, Sun W, Pu H, Zhao JX. Polymer nanoparticles based nano-fluid for enhanced oil recovery at harsh formation conditions. Fuel. 2020; 267 :117251. [ Google Scholar ]
- Zhu L, Tong L, Zhao N, Li J, Lv Y. Coupling interaction between porous biochar and nano zero valent iron/nano α-hydroxyl iron oxide improves the remediation efficiency of cadmium in aqueous solution. Chemosphere. 2019; 219 :493–503. [ PubMed ] [ Google Scholar ]
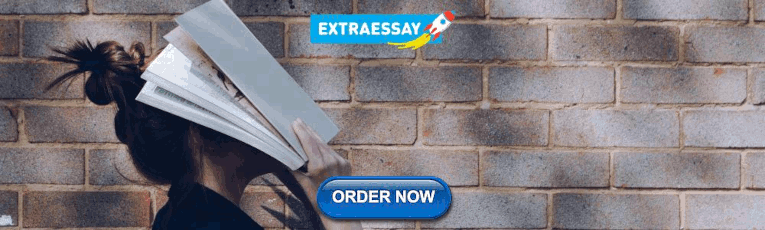
IMAGES
VIDEO
COMMENTS
Nanotechnology is starting to play a role in a number of commercial products, though in an evolutionary, rather than revolutionary way, says Peter Dobson. Peter Dobson Thesis 08 Nov 2016
Nanotechnology, the emerging field that encompasses knowledge from multiple disciplines including chemistry, physics, engineering, and biology, implicates the development and modification of ...
Abstract. Nanotechnology is the innovatory technology of the twenty-first century, and nanoscale materials have created a considerable amount of attention from researchers. It is an emerging interdisciplinary area of research wherever groupings of atoms as well as molecules are handled at the nanometer levels.
Nano-Selenium: Novel Formulations for Biological and Environmental Applications. Description: This dissertation demonstrates, in multiple ways, that nanotechnology can be used to engineer effective solutions. This dissertation employs the colloidal route for synthesis of elemental ….
Nanotechnology, contrary to its name, has massively revolutionized industries around the world. This paper predominantly deals with data regarding the applications of nanotechnology in the modernization of several industries. A comprehensive research strategy is adopted to incorporate the latest data driven from major science platforms. Resultantly, a broad-spectrum overview is presented which ...
Master-thesis in nanotechnology: theory. Supervisor: Associate professor Jacob Linder ([email protected]) The successful applicant is a highly motivated and ambitious student with very good grades. There may be possibilities for excellent students to continue with a Ph.D degree and also to publish their master-thesis
Nanotechnology is an excellent example of an emerging technology, offering engineered nanomaterials with the great potential for producing products with substantially improved performances. 13 Currently, nanomaterials find commercial roles in scratch-free paints, surface coatings, electronics, cosmetics, environmental remediation, sports ...
Nanotechnology in Electronics, Materials Properties, and Devices: State of the Art and Future Challenges. P. M. Visakh, P. M. Visakh. Faculty of Electronic Engineering, Department of Physical Electronics, TUSUR University, Tomsk, 634050 Russia. Search for more papers by this author.
Thesis 27 Apr 2020 Notes on environmental nanoscience Although we seem to understand how nanoscience can impact the environment, we seem to be far off using nanotechnology for environmental ...
Thesis (5) Year. 2020 (218) All; ... This Review describes the nanotechnology-based approaches available to target dendritic cells and induce tolerogenic properties, highlighting applications in ...
The Greek prefix "nano" is derived from the word "dwarf" and refers to a reduction in size, or time, of 10 − 9, which is 1000 times smaller than a micron [15].The nanometer (nm) scale is typically defined as one billionth of a meter or three to five atoms in width—equivalent to 10 Å or smaller than one tenth of a micrometer in at least one dimension.
Dr. Chuanzhen Zhao with Prof. Fabrizio Lombardi, President of the IEEE Nanotechnology Council. The award, bestowed annually, acknowledges a Ph.D. thesis in nanotechnology that demonstrates remarkable technological innovation or excellence. Zhao was selected as the sole recipient for this year's award. In addition to the esteemed recognition ...
Nanomaterials in Tissue Engineering. G. Devanand Venkatasubbu. Ramachandran Chelliah. Bharath Govindan. 282 views. An interdisciplinary journal across nanoscience and nanotechnology, at the interface of chemistry, physics, materials science and engineering. It focuses on new nanofabrication methods and their ap...
Course content. The master's thesis is a project work that gives 30 credits. The project will usually be part of an ongoing research project related to nanomedicine / nanotechnology at the Faculty of Medicine and Health Sciences.
Nanotechnology is a group of evolving technologies with deliberate and controlled manipulation, precision modeling and production of matter at nanoscale (Fig 1.1) in order to create materials, devices and systems with fundamentally new properties and function/s that are not displayed by their larger counterparts. Nanotechnology has created a ...
The author of the best PhD thesis in nanotechnology must have graduated within the past 3 years, and not be advised by a voting member of the NTC Executive Committee (ExCom) or a member of the Best PhD Awards Evaluation Committee. A thesis can be nominated for this award only once. Unsuccessful nominations cannot be carried over or re-submitted ...
In my Thesis of April 2019, I reviewed ten publications by some of the early voices on ethics in nanotechnology, dating from 2001 to 2004 1.I reported that those papers presented a spectrum of ...
Nanotechnology is one of the most emerging fields of research within recent decades and is based upon the exploitation of nano-sized materials (e.g., nanoparticles, nanotubes, nanomembranes, nanowires, nanofibers and so on) in various operational fields. Nanomaterials have multiple advantages, including high stability, target selectivity, and plasticity. Diverse biotic (e.g., Capsid of viruses ...
Nanotechnology Master's Degree Admission Requirements. Bachelor's degree in science or engineering with a GPA of 3.0 or a high GRE test score; ... Most programs require 30 credits, which include a thesis or a final project. Keep a GPA of at least 3.0 at all times.
The main goal of this thesis has been to devise a nanotechnology-based strategy and tools to spatially and temporally control biologically-relevant phenomena in-vitro which are important in some fields of medical research. 5 Gastrow, M. "Thinking small : the state of nanotechnology research and development in South Africa." ...
This thesis focuses on the consideration of a question that the advent of what is called nanotechnology makes it increasingly urgent to philosophy in general and the philosophy of science in particular because of the inexistence of the "nanotechnology" stricto sensu, the lack of good definition and the default of something like a ...
If emerging technologies such as nanotechnology are to reach their full potential we need to radically change our approach to risk, argues Andrew D. Maynard. Andrew D. Maynard Thesis | 05 February ...
Professor Ahmad Umar is at the forefront of research in nanoscience and nanotechnology, areas that explore materials at the nanoscale, between 1-100 nanometers. In simpler terms, he's working with incredibly tiny materials to address some of the most pressing challenges facing our environment and energy sectors.
Abstract. Nanotechnology is one of the most emerging fields of research within recent decades and is based upon the exploitation of nano-sized materials (e.g., nanoparticles, nanotubes, nanomembranes, nanowires, nanofibers and so on) in various operational fields. Nanomaterials have multiple advantages, including high stability, target ...