MIT Libraries home DSpace@MIT
- DSpace@MIT Home
- MIT Libraries
- Doctoral Theses
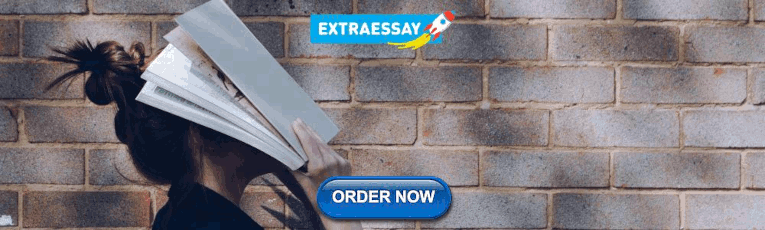
Sustainable cities and institutional change : the transformation of urban stormwater management
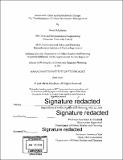
Alternative title
Other contributors, terms of use, description, date issued, collections.
Advertisement
Green IoT for Eco-Friendly and Sustainable Smart Cities: Future Directions and Opportunities
- Open access
- Published: 17 August 2021
- Volume 28 , pages 178–202, ( 2023 )
Cite this article
You have full access to this open access article
- Faris. A. Almalki 1 ,
- S. H. Alsamhi ORCID: orcid.org/0000-0003-2857-6979 2 , 3 ,
- Radhya Sahal 4 , 5 ,
- Jahan Hassan 6 ,
- Ammar Hawbani 7 ,
- N. S. Rajput 8 ,
- Abdu Saif 9 ,
- Jeff Morgan 10 &
- John Breslin 10
24k Accesses
83 Citations
13 Altmetric
Explore all metrics
The development of the Internet of Things (IoT) technology and their integration in smart cities have changed the way we work and live, and enriched our society. However, IoT technologies present several challenges such as increases in energy consumption, and produces toxic pollution as well as E-waste in smart cities. Smart city applications must be environmentally-friendly, hence require a move towards green IoT. Green IoT leads to an eco-friendly environment, which is more sustainable for smart cities. Therefore, it is essential to address the techniques and strategies for reducing pollution hazards, traffic waste, resource usage, energy consumption, providing public safety, life quality, and sustaining the environment and cost management. This survey focuses on providing a comprehensive review of the techniques and strategies for making cities smarter, sustainable, and eco-friendly. Furthermore, the survey focuses on IoT and its capabilities to merge into aspects of potential to address the needs of smart cities. Finally, we discuss challenges and opportunities for future research in smart city applications.
Similar content being viewed by others
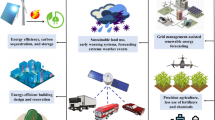
Artificial intelligence-based solutions for climate change: a review
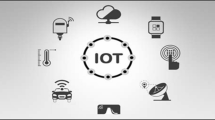
RETRACTED ARTICLE: A Review and State of Art of Internet of Things (IoT)
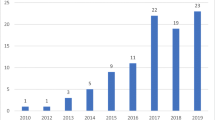
Security, Privacy and Risks Within Smart Cities: Literature Review and Development of a Smart City Interaction Framework
Avoid common mistakes on your manuscript.
1 Introduction
Due to the tremendous development in communication and sensing technologies, ‘things’ around us are being connected together to provide various smart city applications, enhancing our life quality [ 1 ]. This connectivity between things in the smart city is commonly referred to as the Internet of Things (IoT). IoT includes everything in smart cities, to be connected at any time, anywhere, and using any medium [ 2 , 3 ]. The development of IoT technologies continue to grow, making IoT components smarter through an adaptive communication network, processing, analysis, and storage. For context, some IoT devices include cameras, sensors, Radio Frequency Identification (RFID), actuators, drones, mobile phones, etc. All of these have the potential to communicate and work together to reach common goals [ 1 , 4 ]. With such components and communication technologies, IoT devices are set to provide a broad range of applications for real time monitoring, as seen in environmental monitoring [ 5 , 6 ], e-healthcare [ 7 ], transportation autonomy [ 8 ], industry digitalization and automation [ 9 , 10 ] and home automation [ 11 , 12 ]. Furthermore, IoT is an enabler of software Agents, to help share information, make collaborative decisions, and optimally accomplish tasks [ 10 ].
IoT is capable of collecting and delivering vast amounts of data using advanced communication technologies that can be analyzed for intelligent decision making. The Big data requirements of IoT needs storage capacity [ 13 ], cloud computing [ 14 ], and wide bandwidth for transmission, to make IoT ubiquitous. This big processing and transmitting of data consumes high amounts of energy in the IoT devices. However, using efficient and smart techniques could lead to a decrease in power consumption. Therefore, the combination of IoT and the practical techniques to reduce power consumption of big data processing and transmission can improve the quality of life in smart cities, and contribute to making the world greener, more sustainable, and collectively a safer place to live [ 15 , 16 , 17 ]. Shuja et al. summarized this relationship between green IoT and big data to create sustainable, green, and smart cities by decreasing pollution hazards and reducing energy demand and efficient resource utilization [ 18 ].
Presently there is new potential in smart cities to become even smarter than before with the application of advanced technologies, such as Artificial Intelligence (AI). Examples of this can be seen in smart city components including sensor integrated smart transportation systems, cameras in smart monitoring systems, and so on. Vidyasekar et al. [ 19 ] introduced the critical aspects of potential smart cities in 2020, in which things are smarter through smart energy, smart building, smart mobility, smart citizens, smart infrastructure, smart healthcare, smart technology, and smart education and governance. These aspects are shown in Fig. 1 .
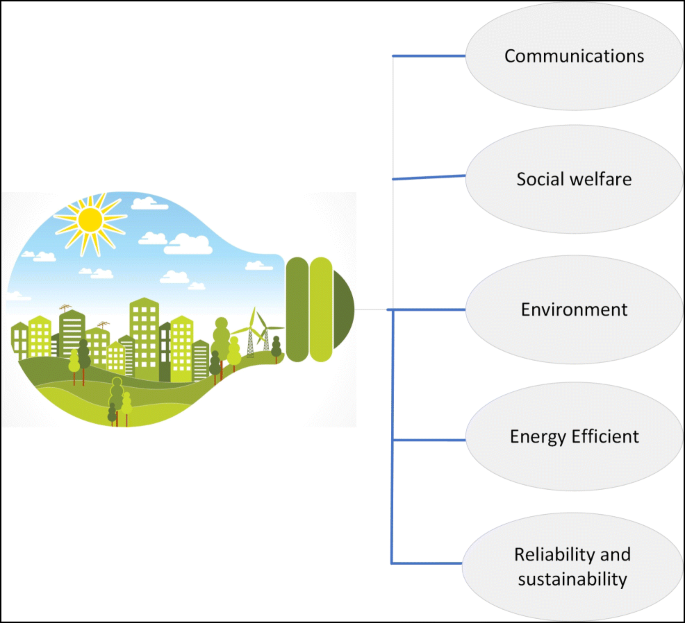
Aspects of smart cities
IoT plays a tremendous role in improving smart cities, affecting in different ways with its numerous applications in enhancing public transformation, reducing traffic congestion, creating cost-effective municipal services, keeping citizens safe and healthier, reducing energy consumption, improving monitoring systems, and reducing pollution, as shown in Fig. 2 . However, IoT environmental issues, such as, energy consumption, carbon emission, energy-saving, trading, carbon labeling and footprint, have attracted researchers’ attention. Therefore, carbon emission reduction and energy efficiency technologies based IoT are summarized [ 20 ]. The study discusses IoT technologies to facilitate real-time intelligent perception of the environment, and generate and collect energy consumption in manufacturing the entire life cycle.
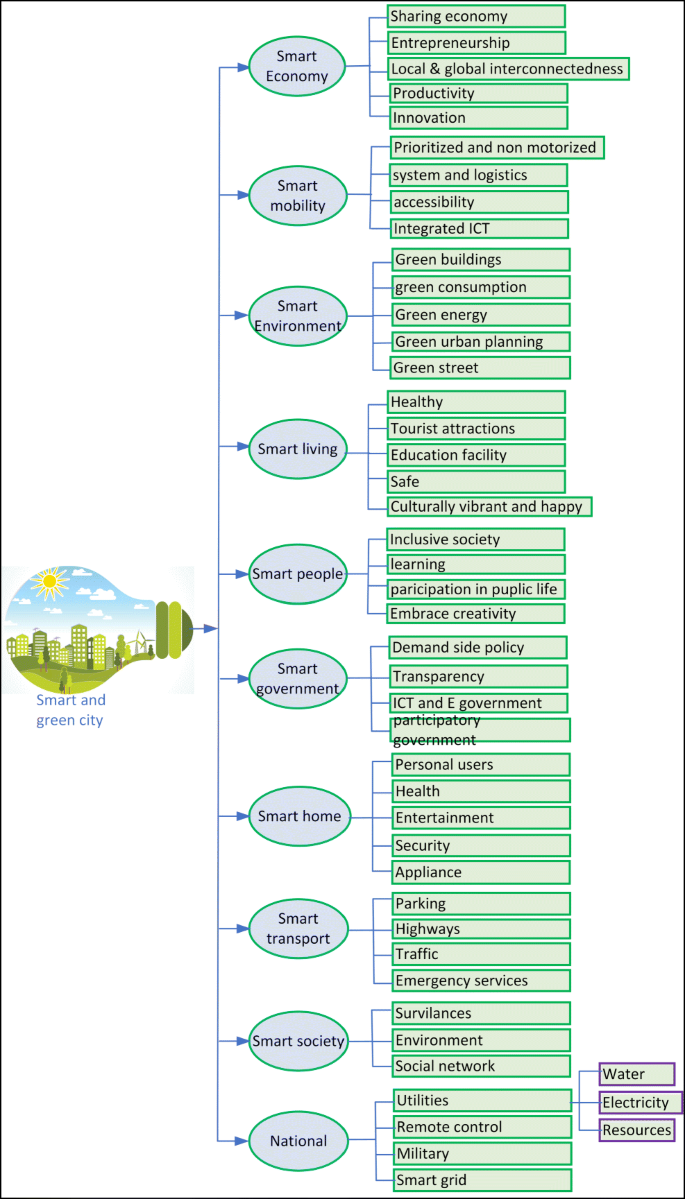
Smart city applications
To fulfill goals of smart cities and sustainability, green IoT is a key technology to decrease carbon emission and power consumption [ 21 , 22 , 23 ]. The increasing number of IoT devices leads to increased energy consumption. For example, wake up protocols and sleep schedules of IoT devices are introduced for energy consumption and resource utilization [ 21 ]. The authors of [ 23 ] provided the techniques that can reduce the energy consumption in IoT via efficient energy of data transmission from IoT devices, data center efficient energy, and design energy-efficient policies. Further, authors in [ 22 ] introduced Information and Communication Technology (ICT) impacts on carbon emissions and smart cities’ energy consumption.
1.1 Related work
The preliminary literature on smart cities based on greening IoT is dispersed [ 23 , 24 , 25 , 26 ], leading to inadequate recognition of the importance of green IoT. There is an apparent lack of depth in current literature which can explain in detail the enabling techniques for IoT systems in smart cities which can reduce C O 2 emission, minimizing power consumption, enhancing QoS [ 27 , 28 , 29 , 30 , 31 , 32 , 33 , 34 , 35 , 36 , 37 , 38 , 39 , 40 ], and enabling ICT. Existing surveys are not comprehensively focusing on smart cities strategies strategies and techniques for enabling greener smart cities. To the best of the authors’ knowledge, there is no existing survey dedicated to reviewing the strategies and techniques for greener smart cities, through enabling ICT, reducing energy consumption, reducing C O 2 emissions, reducing waste management, and improving sustainability.
As a comparison, Arshad et al. [ 23 ] discussed green IoT based on minimizing energy consumption. The study focuses only on designing energy efficient policies, energy efficient policies, energy efficient data centers, and data transmission from IoT devices. However, the study does not cover all of the potential ideas, while our survey will focus on techniques and strategies, for enabling IoT to improve the eco-friendly and sustainability of smart cities. The work presented in [ 25 ] discussed the negative impact of IoT technology and suggested solutions to minimize it. Some negative impacts of IoT were included in this study, e.g., greenhouse gas emissions, and energy usage, etc. The study explored the principles of green IoT to improve life quality, economic growth, and environments in smart cities. It showed evidence that green IoT usage can support sustainable natural resource utilization in agriculture, forestry, and aquaculture. However, the authors did not fully discuss all potential negative impacts of IoT technology in various applications. As such, Our work not only includes a broader coverage of the negative impacts, but also focuses on the use of green IoT to improve eco-friendly and sustainability for smart cities.
In [ 24 ], the authors introduced IoT for smart cities, and addressed techniques for minimizing energy consumption for green IoT, and as such, introduced the green ICT principle. However, the authors did not further discuss the green ICT for IoT applications in smart cities. As such, this paper will fill this gap in the literature. Shaikh et al. [ 26 ] presented how to deploy IoT technology efficiently to fulfill a green IoT. They identified IoT applications where energy consumption can be reduced for a green environment. Several techniques were introduced for enabling green IoT to facilitate energy efficiency. The authors of [ 41 ] discussed the concept of IoT for smart cities and their advantages, benefits, and different applications. The study focused mainly on the use of IoT for smart cities such as smart homes, smart parks, smart transports, weather, and pollution management. The authors focused on the benefits and applications of IoT for smart cities applications, however, the study does not discuss the techniques for improving IoT for enhancing the eco-friendlinesss and sustainability of smart cities. A comparison of existing surveys and the present work is summarized in Table 1 .
1.2 Contribution
This literature review is intended to develop smart cities’ strategies and techniques based on collaborative IoT to improve life quality, sustainability, echo-friendliness, citizen safety, and the health of the environment.This work will contribute to the research literature by broadening discussions on:
Enabling IoT techniques for eco-friendly ICT. Specifically the significant impacts of ICT for reducing energy consumption and C O 2 emissions for a sustainable smart city,
Different strategies and techniques used for energy-efficiency, reduced C O 2 , reduced traffic, and reduced resource usage in smart cities,
Waste management techniques to improve smart cities,
Advanced techniques used for smart city sustainability,
Surveyed current ongoing research works and possible future techniques for smart cities’ sustainability and energy efficiency, based on collaborative IoT.
1.3 The scope of study and structure
In a smart city, IoT plays a critical role in improving the life quality, safe environments, sustainability, and ecosystem. This paper will survey the techniques and strategies used to improve smart cities to be eco-friendly and sustainable. The authors focus on techniques which lead to fewer emissions, reduce traffic, improve waste management, reduce resource usage, reduce energy consumption, reduce pollution and improve Quality of Service (QoS) of communication networks. To the authors’ best knowledge, no previous research work in the survey has addressed the techniques and strategies that lead to eco-friendly and sustainable smart cities. Relevant challenges are addressed, and the solutions are conceived for other purposes, yet related work will be introduced.
The rest of this paper is organized as follows (see Fig. 3 ). ICT technology for smart cities is presented in Section 2 . Section 3 discusses energy efficiency. In Section 4 , reducing pollution hazards is considered. Waste management and sustainability are discussed in Sections 5 and 6 , respectively. The future directions and opportunities are discussed in Sections 7 and 8 , respectively. Finally, we conclude the paper in Section 9 .
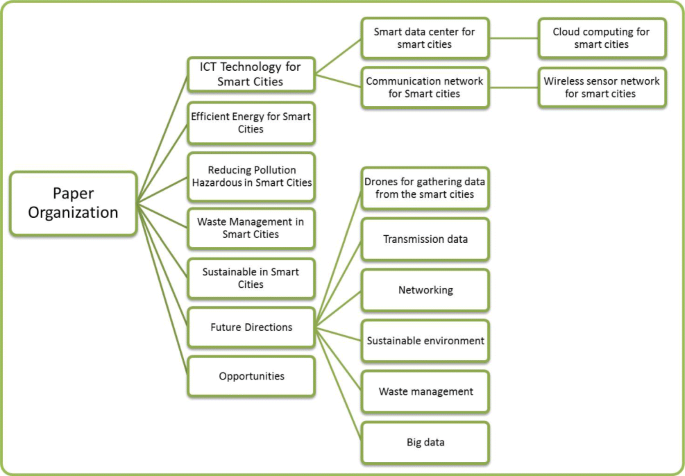
Paper Organization
2 ICT technology for smart cities
IoT is a global, ambient communication network, immersive, and an invisible computing environment built depending on smart sensors, cameras, software, databases, and data centers in smart cities [ 42 ]. In [ 43 ], the authors presented IoT for constructing a green campus environment based on energy efficiency. Despite prior evidence presented in [ 42 ], IoT elements have been presented in [ 4 ], where the benefits of IoT and how to create a green area by employing efficient techniques were discussed. In [ 44 ], the authors discussed different technical directions towards realizing future green Internet.
Consequently, IoT leads to saving natural resources, minimizing the technological impact on the environments and human health, and reducing costs. Thus, green IoT focuses on green manufacturing, green design, green utilization, and green disposal [ 41 ]. The authors in [ 41 ] discussed all of the above categories and their importance for improving smart cities.
Furthermore, Solutions for green IoT includes reducing C O 2 emissions and reducing IoT energy usage to fulfill the smart world with the sustainability of intelligent everything. Green IoT includes designing and leveraging green aspects. The design elements of green IoT include developing computing devices, energy efficiency, communication protocols, and networking architectures [ 45 ]. Leveraging the IoT element is to reduce the emissions of C O 2 , and enhance energy efficiency. Uddin et al. [ 46 ] presented the techniques for improving energy efficiency and reducing C O 2 for enabling green ICT. Gathering data from smart city environments represents the essential element of smart cities that create an intelligent model for appreciated decision making.
ICT plays an essential role in improving green IoT in smart cities to be friendly and sustainable. ICT can reduce cost, resource consumption, and pollution; interact with city services; and enhance life quality. Therefore, without ICT, the idea of smart cities cannot exist. ICT improves the smart cities’ application by automated, simplified, enabling IoT, automatic security threat isolated, and scalability, as shown in Table 2 . Furthermore, ICT technologies can reduce climate change globally [ 42 , 43 , 44 , 47 , 48 ], with ICT application growth with energy efficiency due to environmental awareness. Greening IoT refers to the advanced technologies that make the IoT environmentally friendly by using facilities and storage that enables subscribers to gather, store, access, and manage various information [ 23 ].
Green ICT enables subscribers to gather, access, store, and manage information [ 24 ]. ICTs play a critical role in greening IoT and providing many benefits to society, i.e., saving energy used for designing, manufacturing, and distributing ICT equipment and devices. Various research have been done on green ICT technologies, such as [ 24 , 49 , 50 , 51 , 52 , 53 ]. These are exciting, but they have been applied for limited applications and ways. In [ 49 ], the authors discussed using ICT applications and strategies to reduce C O 2 emissions and energy consumption. The authors [ 50 ] discussed green IoT principles for enhancing life quality, growth, economy, and environment. They provide the numerous benefits of reducing the negative impact of the latest technology on society, human health, and the environment. In the case of stainability, ICT can manage data centers optimization through techniques of sharing infrastructure, which leads to energy efficiency with reduced C O 2 emissions and e-waste of material disposals [ 54 ]. Furthermore, the authors [ 22 ] discussed the enabling technologies for green IoT, which include RFID, wireless sensor networks (WSN), machine to machine (M2M), data center, cloud computing, and communication networks, as shown in Fig. 4 . However, they did not consider the techniques used for greening IoT by reducing energy consumption and C O 2 emissions. Also, the authors [ 51 ] support the idea of [ 24 ] to satisfy greening IoT by transmitting the needful information, reduce the energy consumption of facilities, and use renewable energy sources. Kai et al. [ 53 ] proved that the Device to Device (D2D) communication plays a key technology to make cities greener and smarter. They investigated the combination of power allocation optimization and uplink subcarrier assignment in the D2D underlying cellular networks. Therefore, all users’ power consumption in network was decreased, while guaranteeing the required throughput of both cellular user and device to the device user equipment.
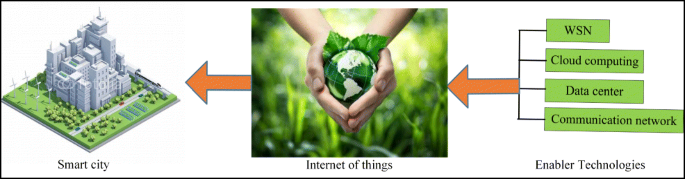
ICT technologies for smart cities
ICT technologies play a vital role in reducing C O 2 emissions and energy consumption to green IoT applications in smart cities, i.e., smart transportation, smart building, smart parking, and so on [ 55 ]. The authors of [ 56 ] described the green ICT and green IoT depending on green smart grid, green communication, and green computing technologies. The benefits of greening enabling IoT are illustrated in Table 3 . It shows the enhancement of green ICT technologies to reduce energy consumption, reduce C O 2 emissions, reduce costs, and change the climate.
Going towards greening IoT involves finding new resources, exploiting environmental conservation, minimizing the use of available resource and costs, and minimizing negative impacts of IoT on human health and environment (e.g., C O 2 emission , N O 2 and other pollution) [ 45 , 57 , 58 , 59 ]. The authors of [ 49 ] provided the details on how industrial emissions influence the environment over time. Therefore, reducing IoT device energy consumption is required to make the environment healthier [ 20 ]. Furthermore, greening ICT technologies help to support environmental sustainability and economic growth [ 45 , 50 ], and therefore, emerging IoT technologies make the world greener and smarter. Table 4 shows the critical trends in IoT for smart cities applications domains such as smart healthcare, smart transportation, smart retail, smart, smart industries, smart house, smart grid, smart agriculture, smart wearable.
2.1 Smart data center for smart cities
Data Center is a repository and technology for smart city management, data storage, and dissemination gathered from smart cities’ devices. A massive number of IoT devices need permanent internet connectivity over the smart city. However, data management and transformation of data into information over a smart city would not be possible without the data center. It consumes a huge amounts of energy [ 22 ], high costs of operation, and high C O 2 footprints due to dealing with different data from different applications. Furthermore, the production of big data is rising through various ubiquitous things, i.e., mobile devices, actuators, sensors, RFID, etc. For the energy efficiency of the data center, the authors of [ 24 , 60 , 61 ] discussed several techniques (i.e., renewable energy, utilizing efficient dynamic power-management, designing more energy-efficient hardware, constructing efficient, designing novel energy-efficient data center architectures, using accurate data center power models, drawing support from communication and computing techniques, and improving air management, consolidating servers, finding optimal environment, improving the processing technology and boost airflow). An eco-friendly datacenter comprises enhancing the airflow and processing, finding optimal environment, improving the air management, and consolidating the server.
Furthermore, the authors of [ 51 ] introduced many techniques for enhancing and predicting the energy efficiency of the data center and its components. In addition to the work of authors [ 51 ], authors in [ 52 , 53 ] presented the optimization technique for the data center energy efficiency with supporting Quality of Service (QoS). The study in [ 62 ] provided a method to reduce the power consumption without degrading the data center cooling efficiency. Peoples et al. [ 63 ] explored the energy-efficient context-aware broker framework mechanisms to manage data center next-generation. However, the study in [ 64 ] offers a green data center of air conditioning via cloud techniques, consisting of two subsystems (i.e., air conditioning in the data center system and cloud management platform). The air conditioning system’s data center includes environmental monitoring, air conditioning, communication, temperature control, and ventilation. Simultaneously, the cloud platform provides data storage, up-layer application, and big data analysis and prediction. Furthermore, an Ant Colony System (ACS) based virtual machine (VM) can be used for reducing the power consumption of the data center while maintaining QoS requirements [ 65 , 66 ] by a near-optimal solution, while virtual machine is considered to reduce the energy consumption of the cloud data center and maintain the desired QoS [ 67 ]. The authors of [ 50 ] discussed the mitigation of VMs for QoS constraints via bandwidth management and minimalizing energy for 5G networks [ 61 ]. Figure 5 illustrates the required impacts for greening the data center for smart cities.
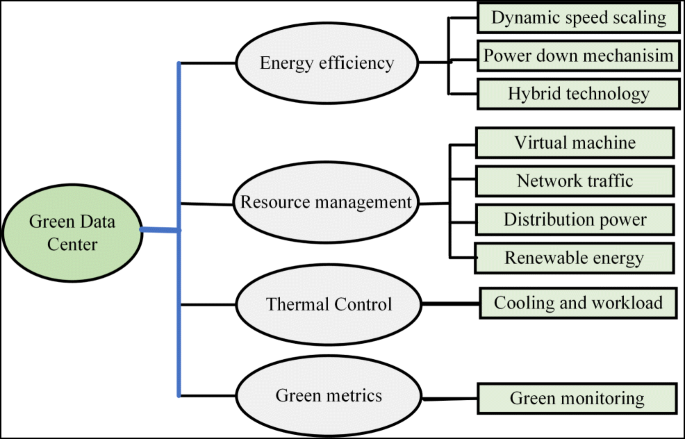
Required impacts for greening the data center
The dynamic speed scaling technique plays a vital role in reducing power consumption, as discussed in details in [ 68 ]. In the case of speed scaling, various researches have addressed signal processing [ 69 ], and network devices [ 70 , 71 ], and parallel processors [56] for saving energy by speed scaling. However, the authors in [ 72 ] combined sleep state and varying the speed when the tasks are processed for reducing energy usage. The study in [ 72 ] supported by Liu et al. [ 73 ], developed SleepScale for power efficiency and fulfilling QoS agreements. In addition to the work of [ 72 , 73 ], the authors in [ 74 ] used hybrid technology to reduce network energy consumption by using idle periods and adapting the rate of network operators to the requested workload.
The authors in [ 75 ] proposed a centralized network power controller based on collected data of traffic. Statistic servers form, and collected data are used to perform the aggregation of transportation and VM assignment, which was used for migrating the target data center. Authors found that the bandwidth and VM reduced the network power consumption for any data center topology. To optimize the power usage in data center networks with guaranteed connectivity and bandwidth utilization, Zhang et al. [ 76 ] discussed two levels for doing the needful. These levels are core level and pod level, in which the purpose of the core level is to define the core switches, while the pod level defines the aggregation switches. They evaluated the hierarchical energy optimization for various traffic patterns, small, large, or random traffic.
Furthermore, the study [ 77 ] focused on reducing energy by two steps:(i) by allocating VM to the server to minimize the traffic amount and (ii) balancing traffic flows by reducing the number of active switches. Zheng et al. [ 78 ] used PowerNets for improving the energy savings of a data center network. The proposed technique gradually improved VM and traffic consolidation performance with lower VM migration overheads by energy savings for a data center.
For power distribution, Meisner et al. [ 79 ] developed a technique to eliminate idle power waste in servers based on the PowerNap and RAILS.The finding showed that both techniques minimized the average power consumption in the server by 74%. Therefore, the proposed methods supported transitioning quickly between near-zero-power idle and high-performance active states in response to immediate load variations. However, the authors in [ 80 ] proposed a method to reduce the utilized power in installing the infrastructure, and they used power routing across redundant power feed for schedule servers.
Renewable energy is another route towards a green data center which minimizes the negative environmental implications. Therefore, Zhang et al. [ 81 ] designed the middleware system to optimize the dynamically distributed requests through various data centers via linear-fractional programming. They found that the proposed system could significantly increase renewable energy usage at different locations without impacting operational cost budges. Furthermore, authors in [ 82 , 83 ] considered the electrical grid and solar array for data center powering. They proposed two schedulers called GreenHadoop and GreenSlot for data processing jobs and parallel batch jobs, respectively. These schedules are used to predict the solar energy amount to maximize the green energy usage. Both schedulers could increase green energy consumption efficiently. Table 5 illustrates the summary of techniques and strategies for energy efficiency, resource management, thermal control, and green metrics for greening data centers.
Availability and sustainability are the factors that can determine the future of data centers. Therefore, smart cities are required for the data center with the high capacity to process big data coming from sensors dispersed in the city. To enhance the technological infrastructure and reduce the cost, the processing of big data needs communication networks, virtualization systems, and storage access. Here, the smart data center will manage the smart cities effectively and efficiently. Therefore, smart data centers represent smart cities’ core, increasing access security, providing passive sensitometry, achieving balanced sustainability, taking care of the city environment, and providing sustainable development for city development. Furthermore, the smart data center will have the capability to effectively and efficiently coordinate and manage the resources required by smart cities. For instance, they are measuring and controlling energy from renewable resources, managing the mobility and traffic, measuring the emissions and pollutions, managing the growth of resources, i.e., air, water, light, ect., and leading other services such as recycling waste, public safety, health, etc. Smart data centers’ future will help create new technologies and architectures for managing smart cities to improve citizens’ quality of life.
2.1.1 Cloud computing for smart cities
Cloud computing is a critical technology for smart cities’ physical infrastructure. The deployment of smart cities requires the combination of a decentralized cloud and a distributed open-source network.Cloud computing services are essential for smart city applications. Therefore, the massive amounts of heterogeneous data collected from different devices surrounding smart cities require the services of cloud computing. Smart cities refer to the high quality of life, management the natural resources, and economic development. Smart cities should intelligently provide the many facilities to improve smart city applications, such as police transport, public safety, security, electric supply, water supply, internet connectivity, smart parking, etc.
Cloud computing provides unlimited computational service delivery via the internet and unlimited storage. It is shown that different devices (i.e., tablet, camera, laptop, mobile, etc.) are connected to gather via the cloud. The combining of cloud computing and IoT together has a comprehensive research scope. The aim of cloud computing is to promote eco-friendly products, which are facilely reused and recycled. Thus, the authors of [ 18 ] proposed green computing with a focus on ICTs. Also, they discussed the trade-off between green computing and high- performance policies. Furthermore, Baccarelli et al. [ 90 ] introduced a green solution to IoT over the fog-supported network.
Therefore, efficient cloud computing plays a vital role in maximizing energy consumption, reducing hazardous materials, and enhancing old products’ recyclability. Moreover, efficient cloud computing achieves product longevity resource allocation and paperless virtualization due to the management of power used. Furthermore, Sivakumar et al. [ 91 ] introduced the integration of IoT and cloud computing in various architectures, applications, protocols, database technologies, service models, and algorithms.
Further, efficient cloud computing plays a vital role in maximizing energy consumption, reducing the use of hazardous materials, and enhancing the recyclability of old products. Moreover, efficient cloud computing achieves product longevity resource allocation and paperless virtualization due to the management of power used. The idea is supported by a study in [ 47 ], which discusses the various technologies for greening cloud computing by reducing energy consumption. It focused on how the combination of cloud and sensors can be used for green IoT agriculture and healthcare domains. Furthermore, Sivakumar et al. [ 91 ] introduced the integration of IoT and cloud computing in various architectures, applications, protocols, database technologies, service models, and algorithms.
Zhu et al. [ 92 ] presented a multi-method data delivery technique for low cost, sensor-cloud (SC) users, and immediate delivery time. Multi-method data delivery includes four kinds of transportation, i.e., delivery from the wireless sensor network to SC users, delivery from cloudlet to SC users, delivery from cloud to SC users, and delivery from SC users to SC users. Minimizing utility power is the main idea of green cloud computing [ 93 ]. Thus, the authors of [ 93 ] introduced the essential technique for improving the data center’s power performance. Private and public clouds required energy consumption in data processing, switching, transmission, and storage [ 94 ]. Table 6 summaries the used techniques and strategies in cloud computing for smart cities.
Despite the numerous works in [ 22 , 81 , 95 , 96 ] which carried out on green cloud computing and provided potential solutions be shown as the adoption of software and hardware for decreasing energy consumption, power-saving using VM techniques, various energy-efficient resource allocation mechanisms and related tasks, and efficient methods for energy-saving systems. The authors in [ 82 ] explored the trade-off of the energy performance for consolidation, which resulted in the desired workload distribution across servers and saves energy. The authors of [ 83 ] summarized the strategies used for economic and green cloud based on multi-tenancy, dynamic provisioning, server utilization, and data center efficiency.
Regarding green cloud computing, the relationships and similarities are discussed between service rate, packet arrival rate, and response time for efficiency improvement in power cost and server utilization [ 97 ]. However, a VM scheduling algorithm plays a vital role in greening cloud computing, which leads to energy consumption minimization [ 98 , 99 ]. In the case of [ 98 ], a machine algorithm is used for migration of loads of hosts, dynamic voltage frequency scaling, and shutdown of underutilized host features. The result of using algorithms led to improving power consumption. Cloud computing availability in smart cities could help ease big data storage, transforming in real-time data processing, and analyzing in real-time. Therefore, cloud computing will enhance speed, sharpness, and cost savings by providing network access on demand for sharing computing resources, which can be scaled as required and rapidly provisioned. The combination of IoT and cloud computing plays a vital role in healthcare applications such as disease prediction intelligently in smart cities [ 100 ].
Furthermore, [ 101 ] presented an intelligent model for healthcare services in smart cities using parallel particle swarm optimization and particle swarm optimization. The proposed model solves task scheduling, reduce medical requests execution time, and maximize medical resources utilization. The economic benefits and costs were discussed in [ 102 ] based on the combination of AI, cloud computing, and IoT. The authors of [ 91 ] proposed fog, cloud, and IoT to mitigate processing loads, reduce cost and time.
2.2 Communication network for smart cities
Greening wireless communication technologies play a crucial role in making IoT greener. Green communications refer to sustainable, energy-efficient, energy-aware, environmentally aware communications. The idea of a green communication network is referring to low C O 2 emissions, low radiation exposure, and low energy consumption. In [ 103 ], the authors proposed a genetic algorithm optimization for the network planning, where the finding showed significantly C O 2 reduction cost and low radiation exposure. The idea supported by a study in [ 104 ], discussed how to maximize the data rate, minimize C O 2 emissions in cognitive WSNs. In addition to the work of authors [ 103 , 104 ], Chan et al. [ 105 ] provided several models to evaluate the use-phase power consumption and C O 2 emissions of wireless telecommunication networks. The designing of Vehicular Ad hoc NETworks (VANETs) was proposed to decrease energy consumption [ 106 ].
The investigation of the energy efficiency in 5G based mobile communication networks are presented in three aspects, i.e., theory models, application, and technology developments [ 107 ]. Furthermore, Abrol et al. [ 108 ] showed the influence and the growing technologies supporting the energy efficiency of Next Generation Networks (NGN) technology. The need for adopting energy efficiency and C O 2 emission is to increase capacity, enhance data rate, and improve QoS of the NGN. Several researchers have addressed solar for saving energy and enhancing QoS, such as [ 27 , 39 , 109 , 110 , 111 , 112 ], reliable storage for saving energy [ 113 ]. Furthermore, the stochastic geometry approach is applied to achieve energy efficiency and maintaining QoS [ 114 ].
Moreover, the utility-based adaptive duty cycle algorithm proposed to reduce delay, increase energy efficiency, and keep a long lifetime [ 115 ]. However, the hypertext transfer protocol was applied to minimize delay and enhance the lifetime for providing reliability [ 116 ]. The development of wireless communication will improve a next-generation network’s performance according to the requirements based on decreasing energy usage, reducing the emission of C O 2 for providing a healthy environment, and green cities.
5G focuses on reducing energy utilization and results to green communication with healthy environments. In 2020, the prediction of green communication is observed that all communication devices and objects will communicate effectively and efficiently using smart and green techniques for a healthy and green life. 5G technology is essential for enhancing the reliability and improving QoS of communication among machines and humans. Also, 5G technology supports a large area’s connectivity, reduces latency, saves energy, and provides higher data rate. The services of 5G for our society are including robotics communication, e-health, interaction human and robotics, media,transport and logistics, e-learning, public safety, e-governance, automotive and industrial systems, etc.[ 117 , 118 , 119 , 120 ].
Many techniques have been used for energy harvesting and energy-efficient methods discussed in [ 121 ]. Regarding energy-saving methods, Wang et al. [ 122 ] proposed a resource allocation approach for minimizing the network’s energy rate. Maximizing the power-efficiency was by relay station with subcarrier for an orthogonal frequency division multiple access. However, the energy efficiency was optimized by using an energy-efficient incentive resource allocation technique for enhancing the cooperation of communication networks [ 123 ], in which the combination of genetic and water drops method for improving energy consumption effectively and efficiently.
Regarding harvesting energy, many studies focus on greening the communication network based on harvested energy, such as [ 124 , 125 , 126 ]. In [ 124 ], the authors focused on resource allocation techniques used for maximizing the energy efficiency of the green cognitive radio network. Furthermore, Ge et al. [ 125 ] discussed the cognitive radio network secured based on multiple-input single-output using to minim transmit the information signal’s power. However, Zheng et al. [ 126 ] introduced the smart grid’s performance and power consumption based on analyzing IEEE802.11ah. The authors [ 127 ] introduced different techniques for greening communication networks in term of energy-efficiency metrics. The power consumption of the network equipment has taken into account transparency and accuracy [ 128 ]. Yang et al. [ 129 ] differentiated renewable and non-renewable energy for green internet routing. However, Hoque et al. [ 130 ] examined techniques to enhance mobile hand-held devices’ energy efficiency. Table 7 summaries the used techniques and strategies in a communication network for smart cities.
2.2.1 Wireless sensor network for smart cities
The combination of sensing and wireless communication has led to WSNs. WSNs have been used in many applications such as fire detection [ 132 , 133 , 134 ], object tracking [ 135 , 136 , 137 ], environmental monitoring [ 138 , 139 , 140 , 141 , 142 ], evolving constraints in the military [ 143 ], control machine health, and monitoring industrial process [ 121 ]. WSNs represent the critical technology that has made IoT flourish. A sensor combines an enormous number of small, low-power, and low-cost electronic devices [ 139 ]. WSN components are including base stations or sinks and a large number of sensors nodes. The sensor node consists of communication unit, sensing unit, processing unit, and power unit [ 139 ]. Sensor nodes are used to measuring global and local environments such as pollution, weather, healthcare, agricultural fields, and so on. Sensors also communicate via wireless channels and deliver the nearest base station’s sensory data using ad-hoc technology. The authors of [ 144 , 145 ] introduced sleep mode for saving sensor power for a long time and supporting green IoT. For energy conservation of WSNs, Khalil et al. proposed the nearest most used routing algorithm, in which the nearest node is active (transmit and receive data), and the rest of the nodes are in sleep mode and keep sensing in idle mode [ 146 ] . Therefore, any node wanting to send data to another node, it will wake up all the nodes along to its roots and then send data accordingly.
Consequently, when the sending data finished, all the nodes will be reset to sleep mode. Sensors can utilize energy harvested directly from the environment, such as the sun, vibrations, kinetic energy, temperature differentials, etc. [ 147 , 148 , 149 , 150 , 151 , 152 ]. Also, the combination of WSN and energy harvested technologies plays a vital role in the green world [ 153 ], on account of energy harvesting is cost comparable with long batteries life. Many techniques are enabling sensor networks for green IoT, such as sensing selection [ 154 ], energy overheads for context-aware sensing [ 155 ], and sleeping schedule [ 156 ] to save energy, reduce the communication delay between sensors nodes.
Battery power is considered the most critical resource in WSN that directly influences network lifetime. Thus, the main goal is to reduce energy consumption and contribute reliable/robust transmission without compromising the overall QoS [ 203 ]. The idea of energy efficiency is supported by Mehmood et al. [ 157 ], which introduced routing protocols for energy efficiency. Similarly, Rani et al. [ 158 ] discussed flexible IoT and the designing hierarchical network’s energy-efficiency. In addition to [ 58 , 157 ], the authors in [ 159 ] introduced green WSN to improve routing and lifetime of WSN. However, the authors of [ 158 ] discussed green WSN for enabling greening IoT based on increasing energy efficiency, reducing relay nodes, extending the network lifetime, and improving the system budget.
Furthermore, the authors of [ 160 ] investigated a cooperative approach to save energy for greening WSNs. A collaborative approach is based on the cluster technique in which multi-hop works as a relay station to ensure the communication between sensors. Furthermore, energy consumption and network resilience provisioning are discussed for enhancing green WSN for fog computing platforms [ 161 ]. Four steps implemented this work: the creation of hierarchical system frameworks, sensor/actuator nodes localization, nodes clustering, creation of optimization model to realize green IoT, and finally the computing the discovering the minimal energy routing path. The results showed that the proposed approach was pliable, energy-saving, and cost-effective. Furthermore, it applies to the different type of IoT applications such as smart city and smart farming applications.
Mahapatra et al. [ 162 ] introduced wake-up radio, error control coding, wireless energy harvesting to enhance the performance of green WSNs while minimizing the C O 2 emissions. Furthermore, the combination of WSN and cloud computing leads to a decrease in demanded high power consumption and C O 2 emission, which significantly affects the environment [ 163 ]. A balanced tree-based WSN is designed for network lifetime maximization and reduces sensor nodes’ energy consumption [ 164 ]. However, the green cooperative cognitive radio was proposed in WSN [ 104 ]. Also, Araujo et al. [ 165 ] proposed cognitive WSN for reducing a large amount of power. Their work was demonstrated and evaluated in three scenarios to enable the development of power reductions and green protocols for cognitive WSN. Regarding green WSN, the following techniques could be adopted [ 22 , 95 , 116 , 166 ] such as sleep and active sensor nodes to save energy consumption, energy depletion, optimization of radio techniques, data reduction mechanisms and energy-efficient routing techniques, hybrid transmission protocol to maximize lifetime reliability. Table 8 summarizes the used techniques and strategies in WSN for smart cities.
Smart cities are recently suffering from several problems such as traffic, pollution, waste management, and high energy consumption. The rapid development and sustainability solutions demand increasing mobility in order to improve environmental impacts. The authors [ 167 ] introduced smart mobility with autonomous vehicles and connected and discussed smart cities’ challenges. The advantages of mobility for enhancing smart cities’ sustainability are discussed [ 168 ], including increasing people’s safety, reducing noise pollution, reducing pollution, improving transfer speed, reducing traffic, and reducing the transferring costs. Furthermore, [ 169 ] discussed how information shared with IoT help in a sustainable value chain network.
3 Efficient energy for smart cities
The drone plays an essential role in greening IoT. It provides efficient energy utilization and hence reducing IoT device’s power consumption. For sending data over long distances, IoT devices need high transmission power. Therefore, the drone can move towards closer to IoT devices to collect data, processing data, and sending data to another device that is in another place. Authors in [ 170 ] introduced a genetic algorithm for improving drone-assisted IoT devices based on energy consumption, sensor density, fly risk level, and flight time. Furthermore, Mozaffari et al. [ 171 ] evaluated the optimal values for small drone cells’ altitude, which leads to the maximum coverage area and minimum transmit power.
Processing in each machine is the primary object of IoT equipment. Drone-equipped IoT devices are used to capture data, process, analyze, manage, storge, and deliver to the cloud. The combination of drones and WSN was discussed [ 172 ]. The framework of drone and WSN is composed of sensor nodes, fixed-group leaders, and drone-Sink. The finding was that the election process and energy consumption were reduced. The techniques of drone-based WSN for data collection were discussed [ 173 ]. The used procedures were able to reduce flying time, energy consumption, and latency of data collection. The authors in [ 174 ] introduced an algorithm for data collection of WSNs by using mobile agents and drones. Therefore, drones and mobile agents are contributed to save time and reduce sensor nodes’ energy consumption. Also, Zorbas et al. [ 175 ] developed a mathematical model for the energy efficiency of IoT devices. The developed model’s performance detects the events that happened on the ground with minimizing power consumption in the coverage area. Furthermore, Sharma et al. [ 176 ] introduced drones’ cooperation with WSN to provide energy-efficient relaying for a better life.
The power needed for a drone is found that energy-efficient components in emerging technologies can improve the energy efficiency [ 177 ]. Choi et al. [ 178 ] formulated the drone efficient energy based relaying by taking into consideration the traffic load and speed factors. On the other hand, the wired drone docking system was developed to perform several functions via the collaboration of drone and IoT devices for reducing wasted resources, reducing energy consumption, and ensuring transmission security [ 179 ]. Moreover, Seo et al. [ 180 ] proposed drones for IoT monitoring, security platform, and emergency response in buildings by utilizing beacons.The authors in [ 181 ] developed an automatic battery replacement mechanism of drone battery lifetime. An automatic battery was used in drones to operate without battery manual replacement.
The selection of the shortest path for packet transmission plays an important role in conserving energy and high efficiency. Engergy 4.0 fault diagnosis framework was presented based on wind turbines [ 182 ]. For improving WSN efficiency, intelligent path optimization is proposed to maximize the rate of network utilization and create the shortest routing path [ 183 ]. The proposed method shows significant improvement in traffic load and network utilization rate for enhancing network performance.
Mahapatra et al. [ 184 ] discussed smart homes’ energy management for making sustainable and green smart cities. Furthermore, the authors proposed NN-based Q–learning for efficient energy management in Canadian homes by decreasing the peak load. Big data analytics represents the most critical part of developing smart city applications. IoT devices are intended to improve smart cities, where they are connected to improve life quality. Therefore, authors in [ 185 ] introduced a new protocol QoS –IoT to reduce the delay of collecting big data from sensors nodes in smart cities and enhance energy efficiency. The study in [ 91 ] discusses an essential issue related to IoT devices’ hardware lifespan in smart cities and energy conservation. Table 9 summarizes the techniques and strategies for energy-efficient for smart cities.
4 Reducing pollution hazardous in smart cities
Recently, monitoring air pollution has become the ultimate essential issue in our environment, life and society. Smart sensors are utilized for pollution monitoring. However, their transmission power is limited for sending data in real-time. Therefore, these sensors can be carried by drones, and it will be easy for gathering data and sending to the destination in real-time. Thus, Villa et al. [ 187 ] developed the best way for gas sensors and a particle number concentration monitor onboard a hexacopter. The authors showed that developed drone system was capable of identifying the point source emissions. The study focuses on airflow behavior and evaluates CO, NO, C O 2 , and N O 2 sensors for monitoring the pollution emissions in a particular area. The potential drone applications explore for interacting with sensor devices to perform remote crop monitoring, soil moisture sensing, water quality monitoring, infrastructure monitoring, and remote sensor deployment [ 165 , 188 ]. The greenhouse pollution should also be considered for controlling the gas emission from the greenhouse. Hamilton et al. [ 189 ] introduced a solar-powered drone carried C O 2 sensing integrated with a WSN. The authors of [ 190 ] proposed drone for remote autonomous food safety and quality. Due to the dynamic and flexible deployment, air pollution monitoring has been found suitable as one of many applications [ 191 , 192 ]. Authors in [ 193 ], reviewed the existing techniques for drone monitoring applications. Furthermore, author of [ 194 ] proposed drones equipped with off-the-shelf sensors for tracking tasks, but they ignored the guidance system. To solve this issue, few authors suggested adopting the pollution-based drone control system. It was based on the chemotaxis meta heuristic and PSO technique, which monitors certain areas on the most polluted zones [ 195 ]. Authors [ 196 ] proposed drone equipped Pixhawk Autopilot to control the drone and a Raspberry Pi for storing and sensing environmental pollution data. Furthermore, authors in [ 197 ] developed an efficient drone platform model to monitor multiple air pollutants. Also, Šmídl et al. [ 198 ] developed the idea of autonomously navigated drones for pollution monitoring. Authors remonstrated the applications of the drone platform in air pollution. It was focusing on air pollution profiling of roadside and air pollution episodes in emergency monitoring. Furthermore, Zang et al. [ 199 ] demonstrated experiences in applying drones to investigate water pollution in Southwest China because of low air pressure, high altitude, severe weather, strong air turbulence, and clouds over. Furthermore, the prediction of carbon footprint in ICT sectors was discussed in [ 200 ].
Air pollution is one of the impact of climate change. However, drone technology currently represent the key technology for monitoring air pollution in order to improve life quality in smart cities. It is used for many scenarios to monitor air pollution and predict air pollution.
5 Waste management in smart cities
Smart cities are running to become smarter and greener. Therefore, companies and governments are searching for efficient solutions to maximize the collection level using intelligent techniques and smart devices, i.e., smart sensors, cloud platforms, IoT, etc. Therefore, Gutierrez et al. [ 201 ] introduced intelligent waste collection cyber-physical system for smart cities based on IoT sensing prototype. IoT sensing prototype measures the waste level in trash bins and sends data to the cloud over the Internet for processing and storage. Based on the collected data, the optimization process can efficiently and dynamically manage the waste collection by forwarding the worker’s necessary action. The authors focused on improving the strategies of waste collection efficacy in real-time through ensuring that when the trash bins were full, the workers would collect in real-time, and therefore, the waste overflow was reduced. Thus, IoT has enabled waste monitoring and management solutions in smart cities within the connected sensors implemented in the container.
Moreover, creating a comprehensive system can help to make cities smarter, healthier, and greener. Hence, the smart waste management (SWM) system helps in decision-making and processing, ensuring the employers follow the procedures and enhance waste collection services delivery [ 202 ]. The SWM system was analyzed in the public university, such as Oradea University [ 203 ]. The designed system at Oradea University was to reduce pollution, protect the environment, and encourage recycling. Employing the SWM at Oradea University was significantly enhanced. Moreover, the authors in [ 204 ] presented ICT application for smart management in Europe and Italy’s circular economy. Likewise, The authors in [ 205 , 206 , 207 , 208 ] [ 205 , 206 , 207 , 208 ] discussed SWM includes IoT technology for smart cities application.
The smart city development system is essential for automated waste collection. Companies and governments are looking for an efficient solution for collecting all kinds of waste using smart IoT devices, edge intelligence, cloud, etc. Therefore, designing, implementing, and developing an automated system to collect waste is required to increase usage, storage, and production capacity. IoT can improve automated waste collection systems by providing real-time monitoring and communication with the cloud. Furthermore, the authors in [ 209 ] focused on increasing automated waste collection systems and improved productivity and capacity. They studied how the system could be integrated with the infrastructure of the smart city. Here, IoT allowed real-time monitoring and data collection in real-time and connected with a cloud of the automated waste collection system. IoT plays a vital role in enhancing the system’s performance by connecting devices and processing and analyzing data in real-time. Therefore, the proposed system could monitor the different types of waste in the containers in real-time. The proposed system helped provide the total amount of waste collected in containers, and optimal discharging equipment status, the optimized route for waste discharged storage system status. However, exploring the possibilities of increasing profit and productivity in waste collection architectures can be considered for future work. In [ 210 ], the authors introduced the existing Italian legislation tools that aimed toward sustainable waste management for smart cities. The waste management technique should foresee the hazard level and the quantity reduction of waste for sustainable development in smart cities.
To enhance environmental protection, and achieve increased efficiency, handle waste for sustainable smart cities is required. Many technologies control waste, such as automatic waste collection, recycling rate, route optimization, and renewable energy. In the case of automated waste collection, IoT devices such as sensors that produced alarms in case of the container are filled up and need to be serviced, thus mange the waste efficiently. Furthermore, smart in-vehicle monitoring makes the waste process faster and ensuring driver safety. IoT is the new technology that can be used for waste management and provide an efficient solution in different ways such as IoT software in waste management, cost efficiency, waste collection, and reduce Greenhouse gas emissions. Furthermore, advanced technologies such as AI and IoT have immensely contributed to reducing the cost and complexity of automated waste systems via improving efficiency, productivity, and safety and minimizing environmental impacts. Disposed waste represents a challenge due to health issues.
6 Sustainability in smart cities
Urban planning has become essential for our very survival in the development of sustainable and green smart cities. Maintaining the wellbeing of every citizen and health are significant factors. The areas are integrated with human right down to waste disposal. Levels of obesity are low, and then the citizens mental health is positive. The structure and design of sustainable green cities are directly connected with human health as well as wellbeing. Through smart networking and environmentally friendly habitats ecological resources are examined, maintained, and environmental benefits are immense. These technologies applications are not for making human life healthy only but also healthy trees, wildlife, and plants. Energy-efficient practices are the key in a green sustainable city. The smart and green disposal techniques help curtail the catastrophic dilemma of green-house gas emissions.
Furthermore, water and food have an impact on growing sustainable smart cities. The role of clean water is vital to the economy in smart cities’ development. Integrated advanced technologies play a crucial role in creating the relationship between government, citizens, environment, ecosystems, infrastructure, and resource utilization. Therefore, sustainable and green cities lead to change in technical and social innovations. On the other hand, sustainable and green cities are also referring to green spaces and smart agricultural resources. Renewable resources, reducing the ecological footprint, and reducing pollution are necessary to keep the city smart and green. IoT plays a vital role in improving smart cities to become more livable, resilient, green, and sustainable.
IoT and smart city technology represent the critical key for developing society and improving life quality. A smart city is created on an intelligent framework and complex manner of ubiquitous networks, objects, government, and connectivity to send and receive data. The data gathered in a cloud of smart cities of any application is managed and analyzed accordingly, for decision making based on the available data, and transform action in real-time to improve the way we work and live. The study [ 211 ] finds out an analysis of the smart cities’ role in making sustainable cities. It is mainly focused on air quality, green energy, renewable, energy efficiency, water quality, and environmental monitoring.
Green IoT plays a vital role in smart cities to make it a greener and sustainable place for working and living. Green IoT techniques and technologies achieve good performance in big data analysis, making smart cities significantly safer, smarter, and more sustainable. The authors of [ 212 ] discussed the big data achievements in improving life quality by reducing pollution and utilizing resources more efficiently. For managing resources utilized by IoT for sustainable and green smart cities, the authors of [ 213 ] introduced delay tolerant streaming and hybrid adaptive bandwidth and power techniques during media transmission in a smart city. Furthermore, the authors of [ 214 ] discussed a sustainable green-IoT environment. However, in [ 215 ] the authors presented greening the technologies process for sustainable smart cities by exploring the greening IoT in improving the environment, life quality, and economy while minimizing the negative impact on the environment and human health.
A smart sustainable city uses ICT to improve life quality, the efficiency of urban services and operation, and competitiveness while ensuring that it meets present and future generations’ economic, social, and environmental needs. A sustainable smart city is an innovative city that uses ICT and IoT technologies to improve life quality, service quality, and competitiveness. Furthermore, it ensures meeting the need of the present and future people regarding social, economic, cultural, and environmental aspects. Due to many people shifting to live in urban and smart cities, the energy resource management, sustainability and sharing, and utilities of emerging technologies need further discussion. Furthermore, addressing the requirements are the most important such as optimizing resources management, growth of business potential, environmental impact, and improving peoples’ life quality
7 Future directions
The upcoming cutting edge disruptive technologies with efficient techniques and strategies will change our future ambience to become healthier, smarter, and greener, delivering very high QoS. This tomorrow would be sustainable environmentally, socially, and economically. The following research fields will seek in depth investigation to improvise and optimize existing solutions for improving smart cities more efficiently.
7.1 Drones for gathering data from the smart cities
The drone is a promising technology which can improvise many real-time applications. Drone technology is a promising solution for making IoT green from both IoT power consumption and device recharging points of views. For example, drones will reduce power consumption of the IoT devices by getting closer to the nodes during data gathering, capture pollution data from agricultural farm lands, and support real-time traffic monitoring and mitigation. Therefore, drones will lead to greener IoT at low cost and with high efficiency and penetration. For pollution monitoring, few IoT devices can be carried out as payloads on a drone to capture real-time data from a large area, and cover different areas dynamically, in a time division mode for energy saving and economy in management expenditures.
Drones can contribute directly in reducing E-waste by wirelessly recharging the IoT devices, enhancing their lifetime. This is particularly useful in large IoT deployments wherein replacing batteries in the massive number of IoT devices would be impractical, thus new deployments would be considered resulting in producing E-waste.
7.2 Transmission data
The data transmission from sensors to the mobile cloud is more beneficial. Sensor-cloud model is now integrating the WSN with the mobile cloud. It is an upcoming technology for greening IoT to improve the sustainability of smart cities. Furthermore, a green social network as a service (SNaaS) may improve the system’s energy efficiency, service provisioning, sensor networks, and management of the WSN on the cloud.
7.3 Networking
It may be perceived from literature that attaining outstanding performance and high QoS on the network is the future direction for green IoT. Finding suitable and efficient techniques for improving QoS parameters (i.e., bandwidth, delay, and throughput) can efficiently improve the smart city’s eco-friendliness. Furthermore, researches are required to design IoT networks which help in reducing C O 2 emission and energy usage. The most critical tasks requiring urgent attention for smart and eco-friendly environment include energy efficiency, resource utilization, and C O 2 emission reduction.
7.4 Sustainable environment
While shaping up a sustainable and eco-friendly network environment for future, it will require less energy demand, newer resources and minimization of the negative impact of IoT on the health of the humankind without disturbing the environment. While machines are getting connected to machines via the Internet to reduce energy, smart devices have to be smarter and greener to enable automation in smart city. Therefore, machine based automation delays can be reduced in case of traffic and taking immediate action. Furthermore, during the machine to machine communication, energy balancing is required in which the radio frequency energy harvesting should be taken into consideration.
7.5 Waste management
Briefly, the future directions in waste management can be categorized based on enabling impacts, emerging technologies, and objectives. Waste gathering and recovery infrastructure have to focus on the automatizing process, implement the best practices with values. IoT devices and technologies have received enough attention in the smart cities domain. Waste management and smart communities need to be addressed and defined. In emerging technologies, smart cities propose to use many smart devices based on processing and computing capabilities that support green automation, monitoring and data collection. In enabling factors, planning, society, economics are essential to understand the waste management platform and creating value from the controlled collection and disposal of waste. Furthermore, the waste management and collection of smart city infrastructures should be taken into considerations. The connection between waste management and smart communities’ activities need to be addressed in a coherent manner.
7.6 Big data
The challenge in the accumulated big data is the prediction and estimation of the required energy for analysis of the gathered data. Rapid analysis of big data may be taken into consideration. If the volume of big data increases, it will increase the exponential scale-up of the cost and resources required for the analysis. Hence, big data analytics may be considered to enhance the prediction of energy efficiency versus the improvement of the life quality [202]. Deep learning techniques can be applied to getting accurate estimation for energy efficiency and the ways to reduce it further to meet greener ranges of system design and deployments. Table 10 summaries the comparison of recent studies with suggestion for future improvement.
8 Opportunities
Smart cities’ technologies bring many advantages by using IoT devices such as sensors, actuators, wearable devices. To improve smart cities, autonomous cars with potential services enabled by vehicle to vehicle and vehicle to internet wireless communication is a technology disruption. It will change the ways in which taxies have been run and owned thus far. For example, improving traffic flow and reducing accidents via intelligent systems and collaborative IoT devices will enhance communication with autonomous cars. Furthermore, autonomous vehicles can also get passengers in demand based on loading and unloading areas. Moreover, improving traffic flow can allow public service to optimize evacuation planning in natural disasters [ 225 , 226 , 227 ]. In order to make our life easier, machine learning and IoT devices are necessary for improving efficiency. Smarter waste management, using IoT technology, utilizes the consideration of our waste disposal by data gathered and how much waste is produced to collect data and then use collected data to implement models to reduce waste in the nearest future by recycling and separation. Today, IoT technology plays a vital role in making city cleaner, healthier, and happier citizens. Improving healthcare and quality of life via the monitoring of environment, air quality, and reduce health stress. Therefore, there are many opportunities for prospective future to create a smarter, healthier, greener, and happier citizen, leading to a cleaner, greener planet.
9 Conclusion
Tremendous developments of various technologies in the 21 st century has improved life quality in smart cities. Recently, IoT technology has demonstrated heightened benefits in enhancing our life quality in smart cities. However, the technologies development demands high energy accompanied by unintentional e-waste and pollution emissions. This survey studied the strategies and techniques to improve our life quality by making the cities smarter, greener, sustainable, and safer. In specific, we highlighted the green IoT for efficient resource utilization, creating a sustainable, reducing energy consumption, reducing pollution, and reducing e-waste. This survey provided a practical insight for anyone who wishes to find out research in the field of eco-friendly and sustainable city- based on emerging IoT technologies. Based on the critical factors of enabling technologies, the smart things in smart cities become smarter to perform their tasks autonomously. These things communicate among themselves and humans with efficient bandwidth utilization, energy efficiency, mitigation of hazardous emissions, and reducing e-waste to make the city eco-friendly and sustainable. We also identified the challenges and prospective future research direction in developing eco-friendly and sustainable smart cities.
Atzori L, Iera A, Morabito G (2010) The internet of things: a survey. Comput Netw 54 (15):2787–2805
Article MATH Google Scholar
Minerva R, Biru A, Rotondi D (2015) Towards a definition of the Internet of Things (IoT). IEEE Internet Initiative 1(1):1–86
Google Scholar
Perera C, Zaslavsky A, Christen P, Georgakopoulos D (2014) Context aware computing for the internet of things: a survey. IEEE Commun Surv Tutor 16(1):414–454
Article Google Scholar
Gubbi J, Buyya R, Marusic S, Palaniswami M (2013) Internet of things (iot): a vision, architectural elements, and future directions. Future Gen Comput Syst 29(7):1645–1660
Tellez M, El-Tawab S, Heydari HM (2016) Improving the security of wireless sensor networks in an iot environmental monitoring system. In: Systems and information engineering design symposium (SIEDS) IEEE. IEEE, Conference Proceedings, pp 72–77
Shah J, Mishra B (2016) Iot enabled environmental monitoring system for smart cities. In: Internet of things and applications (IOTA), International conference on. IEEE, Conference Proceedings, pp 383–388
Chen X, Ma M, Liu A (2018) Dynamic power management and adaptive packet size selection for iot in e-healthcare. Comput Electric Eng 65:357–375
Kong L, Khan MK, Wu F, Chen G, Zeng P (2017) Millimeter- wave wireless communications for iot-cloud supported autonomous vehicles: overview, design, and challenges. IEEE Commun Mag 55 (1):62–68
POPA D, POPA DD, CODESCU M-M (2017) Reliabilty for a green internet of things. Buletinul AGIR nr 45–50
Prasad SS, Kumar C (2013) A green and reliable internet of things. Commun Netw 5(01):44
Pavithra D, Balakrishnan R (2015) Iot based monitoring and control system for home automation. In: Communication technologies (GCCT) global conference on. IEEE, Conference Proceedings, pp 169–173
Kodali RK, Jain V, Bose S, Boppana L (2016) Iot based smart security and home automation system. In: Computing, communication and automation (ICCCA) international conference on. IEEE, Conference Proceedings, pp 1286–1289
Gu M, Li X, Cao Y (2014) Optical storage arrays: A perspective for future big data storage. Light Scie Appl 3(5):e177
Hashem IAT, Yaqoob I, Anuar NB, Mokhtar S, Gani A, Khan SU (2015) The rise of big data on cloud computing: Review and open research issues. Inf Syst 47:98–115
Syed F, Gupta SK, Hamood Alsamhi S, Rashid M, Liu X (2020) A survey on recent optimal techniques for securing unmanned aerial vehicles applications. Trans Emerg Telecommun Technol e4133
Alsamhi SH, Ansari MS, Zhao L, Van SN, Gupta SK, Alammari AA, Saber AH, Hebah MYAM, Alasali MAA, Aljabali HM (2019) Tethered balloon technology for green communication in smart cities and healthy environment. In: First international conference of intelligent computing and engineering (ICOICE). IEEE, Conference Proceedings, pp 1–7
Alsamhi SH, Ma O, Ansari MS, Almalki FA (2019) Survey on collaborative smart drones and internet of things for improving smartness of smart cities. Ieee Access 7:128125–128152
Shuja J, Ahmad RW, Gani A, Ahmed AIA, Siddiqa A, Nisar K, Khan SU, Zomaya AY (2017) Greening emerging it technologies: techniques and practices. J Int Serv Appl 8(1):9
Vidyasekar AD (2013) Strategic opportunity analysis of the global smart city market: Smart city market is likely to be worth a cumulative 1.565 trillion by 2020. Frost & Sullivan
Arshad R, Zahoor S, Shah MA, Wahid A, Yu H (2017) Green iot: an investigation on energy saving practices for 2020 and beyond. IEEE Access 5:15667–15681
Khan R, Khan SU, Zaheer R, Khan S (2012) Future internet: The internet of things architecture, possible applications and key challenges. In: Frontiers of information technology (FIT), 10th International Conference on. IEEE, Conference Proceedings, pp 257–260
Zhu C, Leung VC, Shu L, Ngai EC-H (2015) Green internet of things for smart world. IEEE Access 3:2151–2162
Shaikh FK, Zeadally S, Exposito E (2017) Enabling technologies for green internet of things. IEEE Syst J 11(2):983–994
Talari S, Shafie-Khah M, Siano P, Loia V, Tommasetti A, Catalão J (2017) A review of smart cities based on the internet of things concept. Energies 10(4):421
Alsamhi SH, Ma O, Ansari MS, Meng Q (2019) Greening internet of things for greener and smarter cities: a survey and future prospects. Telecommun Syst 72(4):609–632
Zahmatkesh H, Al-Turjman F (2020) Fog computing for sustainable smart cities in the iot era: Caching techniques and enabling technologies-an overview. Sustainable Cities and Society, p 102139
Alsamhi SH, Rajput NS (2015) Implementation of call admission control technique in hap for enhanced qos in wireless network deployment, Telecommun Syst 1–11. [Online]. Available: https://doi.org/10.1007/s11235-015-0108-4
Alsamhi SHA, Rajput NS (2012) Methodology for coexistence of high altitude platform ground stations and radio relay stations with reduced interference. Int J Scientif Eng Res 3:1–7
SH, Ma O, Ansari MS, Gupta SK (2019) Collaboration of drone and internet of public safety things in smart cities: An overview of qos and network performance optimization. Drones 3(1):13
Alsamhi SH, Rajput NS (2014) Neural network in intelligent handoff for qos in hap and terrestrial systems. Int J Mater Sci Eng 2:141–146
Alsamhi SH, Rajput NS (2015) An intelligent hap for broadband wireless communications: developments, qos and applications. Int J Electron Electric Eng 3(2):134–143
Saif A, Dimyati KB, Noordin KAB, Shah NSM, Alsamhi SH, Abdullah Q, Farah N (2021) Distributed clustering for user devices under unmanned aerial vehicle coverage area during disaster recovery. arXiv: 2103.07931
Alsamhi SH, Almalki F, Ma O, Ansari MS, Lee B (2021) Predictive Estimation of Optimal Signal Strength from Drones over IoT Frameworks in Smart Cities. IEEE Transactions on Mobile Computing. IEEE
Alsamhi SH, Rajput NS (2014) Performance and analysis of propagation models for efficient handoff in high altitude platform system to sustain qos. In: IEEE students’ conference on electrical, electronics and computer science. IEEE, Conference Proceedings, pp 1–6
Gupta A, Sundhan S, Alsamhi SH, Gupta SK (2020) Review for capacity and coverage improvement in aerially controlled heterogeneous network. Springer, Berlin, pp 365–376
Gupta A, Sundhan S, Gupta SK, Alsamhi SH, Rashid M (2020) Collaboration of uav and hetnet for better qos: A comparative study. Int J Veh Inf Commun Syst 5(3):309–333
Almalki FA, Angelides MC (2019) Deployment of an aerial platform system for rapid restoration of communications links after a disaster: a machine learning approach. Computing 1–36
Alsamhi SH, Afghah F, Sahal R, Hawbani A, Al-qaness AA, Lee B, Guizani M (2021) Green iot using uavs in b5g networks: A review of applications and strategies, arXiv: 2103.17043
Alsamhi SH (2015) Quality of service (qos) enhancement techniques in high altitude platform based communication networks, Thesis
Al-Samhi S, Rajput N (2012) Interference environment between high altitude platform station and fixed wireless access stations. System 4:5
Nandyala CS, Kim H-K (2016) Green iot agriculture and healthcareapplication (gaha). Int J Smart Home 10(4):289–300
Sala S Information and communication technologies for climate change adaptation, with a focus on the agricultural sector
Eakin H, Wightman PM, Hsu D, Gil Ramón VR, Fuentes-Contreras E, Cox MP, Hyman T-AN, Pacas C, Borraz F, González-Brambila C (2015) Information and communication technologies and climate change adaptation in latin america and the caribbean: A framework for action. Clim Dev 7 (3):208–222
Upadhyay AP, Bijalwan A (2015) Climate change adaptation: services and role of information communication technology (ict) in india. Amer J Environ Protect 4(1):70–74
Gapchup A, Wani A, Wadghule A, Jadhav S (2017) Emerging trends of green iot for smart world. Int J Innov Res Comput Commun Eng 5(2):2139–2148
Uddin M, Rahman AA (2012) Energy efficiency and low carbon enabler green it framework for data centers considering green metrics. Renew Sust Energ Rev 16(6):4078–4094
Zanamwe N, Okunoye A (2013) Role of information and communication technologies (icts) in mitigating, adapting to and monitoring climate change in developing countries. In: International conference on ICT for Africa, Conference Proceedings
Mickoleit A (2010) Greener and smarter: Icts, the environment and climate change. OECD Publishing, Report
Lü Y-L, Geng J, He G-Z (2015) Industrial transformation and green production to reduce environmental emissions: Taking cement industry as a case. Adv Clim Chang Res 6(3):202–209
Radu L-D (2016) Determinants of green ict adoption in organizations: A theoretical perspective. Sustainability 8(8):731
Dayarathna M, Wen Y, Fan R (2016) Data center energy consumption modeling: A survey. IEEE Commun Surv Tutor 18(1):732–794
Cordeschi N, Shojafar M, Amendola D, Baccarelli E (2015) Energy-efficient adaptive networked datacenters for the qos support of real-time applications. J Supercomput 71(2):448–478
Shuja J, Bilal K, Madani SA, Othman M, Ranjan R, Balaji P, Khan SU (2016) Survey of techniques and architectures for designing energy-efficient data centers. IEEE Syst J 10(2):507–519
Di Salvo AL, Agostinho F, Almeida CM, Giannetti BF (2017) Can cloud computing be labeled as green? insights under an environmental accounting perspective. Renew Sust Energ Rev 69:514–526
Gelenbe E, Caseau Y (2015) The impact of information technology on energy consumption and carbon emissions. Ubiquity 2015:1
Ozturk A, Umit K, Medeni IT, Ucuncu B, Caylan M, Akba F, Medeni TD (2011) Green ict (information and communication technologies): A review of academic and practitioner perspectives. Int J eBusiness eGovernment Stud 3(1):1–16
Murugesan S, it Harnessing green (2008) Principles and practices. IT professional 10(1)
Rani S, Talwar R, Malhotra J, Ahmed SH, Sarkar M, Song H (2015) A novel scheme for an energy efficient internet of things based on wireless sensor networks. Sensors 15(11):28603–28626
Huang J, Meng Y, Gong X, Liu Y, Duan Q (2014) A novel deployment scheme for green internet of things. IEEE Internet Things J 1(2):196–205
Baccarelli E, Amendola D, Cordeschi N (2015) Minimum-energy bandwidth management for qos live migration of virtual machines. Comput Netw 93:1–22
Amendola D, Cordeschi N, Baccarelli E (2016) Bandwidth management vms live migration in wireless fog computing for 5g networks. In: Cloud Networking (Cloudnet), 5th IEEE International Conference on. IEEE, Conference Proceedings, pp 21– 26
Roy A, Datta A, Siddiquee J, Poddar B, Biswas B, Saha S, Sarkar P (2016) Energy-efficient data centers and smart temperature control system with iot sensing. In: Information technology, electronics and mobile communication conference (IEMCON), IEEE 7Th Annual. IEEE, Conference Proceedings, pp 1–4
Peoples C, Parr G, McClean S, Scotney B, Morrow P (2013) Performance evaluation of green data centre management supporting sustainable growth of the internet of things. Simul Model Pract Theory 34:221–242
Liu Q, Ma Y, Alhussein M, Zhang Y, Peng L (2016) Green data center with iot sensing and cloud-assisted smart temperature control system. Comput Netw 101:104–112
Farahnakian F, Ashraf A, Pahikkala T, Liljeberg P, Plosila J, Porres I, Tenhunen H (2015) Using ant colony system to consolidate vms for green cloud computing. IEEE Trans Serv Comput 8 (2):187–198
Ashraf A, Porres I (2017) Multi-objective dynamic virtual machine consolidation in the cloud using ant colony system. arXiv: 1701.00383
Matre P, Silakari S, Chourasia U (2016) Ant colony optimization (aco) based dynamic vm consolidation for energy efficient cloud computing. Int J Comput Sci Inform Secur 14(8):345
Jin X, Zhang F, Vasilakos AV, Liu Z (2016) Green data centers: A survey, perspectives, and future directions. arXiv: 1608.00687
Bansal N, Kimbrel T, Pruhs K (2007) Speed scaling to manage energy and temperature. J ACM (JACM) 54(1):3
Article MathSciNet MATH Google Scholar
Andrews M, Anta AF, Zhang L, Zhao W (2012) Routing for power minimization in the speed scaling model. IEEE/ACM Trans Netw 20(1):285–294
Bampis E, Kononov A, Letsios D, Lucarelli G, Sviridenko M (2018) Energy-efficient scheduling and routing via randomized rounding. J Sched 21(1):35–51
Irani S, Shukla S, Gupta R (2007) Algorithms for power savings. ACM Trans Algo (TALG) 3(4):41
Liu Y, Draper SC, Kim NS (2014) Sleepscale: runtime joint speed scaling and sleep states management for power efficient data centers. In: Computer Architecture (ISCA), ACM/IEEE 41st International Symposium on. IEEE, Conference Proceedings, pp 313–324
Nedevschi S, Popa L, Iannaccone G, Ratnasamy S, Wetherall D (2008) Reducing network energy consumption via sleeping and rate-adaptation. In: NsDI, vol 8. pp 323–336
McGeer R, Mahadevan P, Banerjee S (2010) On the complexity of power minimization schemes in data center networks. In: IEEE global telecommunications conference GLOBECOM 2010 Conference Proceedings, pp 1–5
Zhang Y, Ansari N (2015) Hero: Hierarchical energy optimization for data center networks. IEEE Syst J 9(2):406–415
Wang L, Zhang F, Aroca JA, Vasilakos AV, Zheng K, Hou C, Li D, Liu Z (2014) Greendcn: a general framework for achieving energy efficiency in data center networks. IEEE J Select Areas Commun 32(1):4–15
Zheng K, Wang X, Li L, Wang X (2014) Joint power optimization of data center network and servers with correlation analysis. In: INFOCOM, Proceedings IEEE. IEEE, Conference Proceedings, pp 2598–2606
Meisner D, Gold BT, Wenisch TF (2009) Powernap: eliminating server idle power. SIGARCH Comput Archit. News 37(1):205– 216
Pelley S, Meisner D, Zandevakili P, Wenisch TF, Underwood J (2010) Power routing: dynamic power provisioning in the data center. In: ACM Sigplan Notices, vol 45. ACM, Conference Proceedings, pp 231–242
Sarathe R, Mishra A, Sahu SK (2016) Max-min ant system based approach for intelligent vm migration and consolidation for green cloud computing. Int J Comput Appl 136(13)
Srikantaiah S, Kansal A, Zhao F (2008) Energy aware consolidation for cloud computing
Kumar S, Buyya R (2012) Green cloud computing and environmental sustainability. In: Murugesan S, Gangadharan GR (eds) Harnessing green it. https://doi.org/10.1002/9781118305393.ch16
Greiner G, Nonner T, Souza A (2014) The bell is ringing in speed-scaled multiprocessor scheduling. Theor Comput Syst 54(1):24–44
Van HN, Tran FD, Menaud J-M (2009) Sla-aware virtual resource management for cloud infrastructures. In: 9th IEEE international conference on computer and information technology (CIT’09). Conference Proceedings, pp 1–8
Li C, Jian S, Min Z, Qi P, Zhe H (2019) Multi-scenario application of power iot data mining for smart cities. In: Proceedings of Purple Mountain Forum-international forum on smart grid protection and control. Springer, Conference Proceedings, pp 823–834
Goiri I, Le K, Nguyen TD, Guitart J, Torres J, Bianchini R (2012) Greenhadoop: leveraging green energy in data-processing frameworks. In: Proceedings of the 7th ACM european conference on computer systems. ACM, Conference Proceedings, pp 57–70
Zhang Y, Wang Y, Wang X (2011) Greenware: Greening cloud-scale data centers to maximize the use of renewable energy. In: ACM/IFIP/USENIX international conference on distributed systems platforms and open distributed processing. Springer, Conference Proceedings, pp 143–164
Bhatt JG, Jani OK, Bhatt CB (2020) Automation based smart environment resource management in smart building of smart city. Springer, Berlin, pp 93–107
Baccarelli E, Naranjo PGV, Scarpiniti M, Shojafar M, Abawajy JH (2017) Fog of everything: Energy-efficient networked computing architectures, research challenges, and a case study. IEEE Access
Deep B, Mathur I, Joshi N (2020) An approach toward more accurate forecasts of air pollution levels through fog computing and IoT. Springer, Berlin, pp 749–758
Zhu C, Leung VC, Wang K, Yang LT, Zhang Y (2017) Multi-method data delivery for green sensor-cloud. IEEE Commun Mag 55(5):176–182
Garg SK, Buyya R (2012) Green cloud computing and environmental sustainability. Harnessing Green IT: Principles and Practices 315–340
Chen F, Schneider J, Yang Y, Grundy J, He Q (2012) An energy consumption model and analysis tool for cloud computing environments. In: First international workshop on green and sustainable software (GREENS). Conference Proceedings, pp 45–50
Shaikh FK, Zeadally S, Exposito E (2015) Enabling technologies for green internet of things. IEEE Systems Journal
Liu X-F, Zhan Z-H, Zhang J (2017) An energy aware unified ant colony system for dynamic virtual machine placement in cloud computing. Energies 10(5):609
Peoples C, Parr G, McClean S, Morrow P, Scotney B (2013) Energy aware scheduling across ’green’cloud data centres. In: Integrated Network Management (IM 2013), IFIP/IEEE International Symposium On. IEEE, Conference Proceedings, pp 876– 879
Lago DGd, Madeira ER, Bittencourt LF (2011) Power-aware virtual machine scheduling on clouds using active cooling control and dvfs. In: Proceedings of the 9th International Workshop on Middleware for Grids, Clouds and e-Science. ACM, Conference Proceedings, p 2
Cotes-Ruiz IT, Prado RP, García-Galán S, Muñoz-Expósito JE, Ruiz-Reyes N (2017) Dynamic voltage frequency scaling simulator for real workflows energy-aware management in green cloud computing. PloS One 12(1):e0169803
Abdelaziz A, Salama AS, Riad AM, Mahmoud AN (2019) A machine learning model for predicting of chronic kidney disease based internet of things and cloud computing in smart cities. Springer International Publishing, Cham, pp 93–114. [Online]. Available: https://doi.org/10.1007/978-3-030-01560-2_5
Abdelaziz A, Salama AS, Riad AM (2019) A swarm intelligence model for enhancing health care services in smart cities applications. Springer, Berlin, pp 71–91
Mishra KN, Chakraborty C (2020) A Novel Approach Toward Enhancing the Quality of Life in Smart Cities Using Clouds and IoT-Based Technologies. Springer International Publishing, Cham, pp 19–35. [Online]. Available: https://doi.org/10.1007/978-3-030-18732-3_2
Koutitas G (2010) Green network planning of single frequency networks. IEEE Trans Broadcast 56(4):541–550
Naeem M, Pareek U, Lee DC, Anpalagan A (2013) Estimation of distribution algorithm for resource allocation in green cooperative cognitive radio sensor networks. Sensors 13(4):4884– 4905
Chan CA, Gygax AF, Wong E, Leckie CA, Nirmalathas A, Kilper DC (2012) Methodologies for assessing the use-phase power consumption and greenhouse gas emissions of telecommunications network services. Environ Sci Technol 47(1):485–492
Feng W, Alshaer H, Elmirghani JM (2010) Green information and communication technology: energy efficiency in a motorway model. IET Commun 4(7):850–860
Mao G (2017) 15g green mobile communication networks. China Commun 14(2):183–184
Abrol A, Jha RK (2016) Power optimization in 5g networks: a step towards green communication. IEEE Access 4:1355– 1374
Alsamhi SH, Rajput NS (2016) An efficient channel reservation technique for improved qos for mobile communication deployment using high altitude platform. Wirel Pers Commun 1–14. [Online]. Available: https://doi.org/10.1007/s11277-016-3514-3
Alsamhi S, Rajput NS (2015) An intelligent hand-off algorithm to enhance quality of service in high altitude platforms using neural network. Wirel Pers Commun 82(4):2059–2073. [Online]. Available: https://doi.org/10.1007/s11277-015-2333-2
Alsamhi S, Rajput NS (2014) Hap antenna radiation pattern for providing coverage and service characteristics. In: Advances in computing, communications and informatics (ICACCI), international conference on conference proceedings, pp 1434– 1439
Alsamhi SH, Ma O (2017) Optimal technology for green life and healthy environment, Disaster medicine and public health preparedness, vol Communicated
Li J, Liu Y, Zhang Z, Ren J, Zhao N (2017) Towards green iot networking: Performance optimization of network coding based communication and reliable storage. IEEE Access
Zhou L, Sheng Z, Wei L, Hu X, Zhao H, Wei J, Leung VC (2016) Green cell planning and deployment for small cell networks in smart cities. Ad Hoc Netw 43:30–42
Wang J, Hu C, Liu A (2017) Comprehensive optimization of energy consumption and delay performance for green communication in internet of things. Mobile Information Systems, vol. 2017
Liu A, Zhang Q, Li Z, Choi Y-J, Li J, Komuro N (2017) A green and reliable communication modeling for industrial internet of things. Comput Electric Eng 58:364–381
Sahal R, Alsamhi SH, Breslin JG, Ali MI (2021) Industry 4.0 towards forestry 4.0: Fire detection use case. Sensors 21(3):694
Alsamhi SH, Lee B, Guizani M, Kumar N, Qiao Y, Liu X (2021) Blockchain for decentralized multi-drone to combat covid-19 and future pandemics: Framework and proposed solutions. Trans Emerg Telecommun Technol e4255
Sahal R, Alsamhi SH, Breslin JG, Brown KN, Ali MI (2021) Digital twins collaboration for automatic erratic operational data detection in industry 4.0. Appl Sci 11(7):3186
Alsamhi SH, Lee B (2020) Block-chain empowered multi-robot collaboration to fight covid-19 and future pandemics. IEEE Access
Wu Y, Zhou F, Li Z, Zhang S, Chu Z, Gerstacker WH (2018) Green communication and networking. Wirel Commun Mob Comput 2018
Wang T, Ma C, Sun Y, Zhang S, Wu Y (2018) Energy efficiency maximized resource allocation for opportunistic relay-aided ofdma downlink with subcarrier pairing. Wirel Commun Mob Comput 2018
Liu ZY, Mao P, Feng L, Liu SM (2018) Energy-efficient incentives resource allocation scheme in cooperative communication system. Wirel Commun Mob Comput 2018
Yang Z, Jiang W, Li G (2018) Resource allocation for green cognitive radios: Energy efficiency maximization. Wirel Commun Mob Comput 2018
Ge W, Zhu Z, Wang Z, Yuan Z (2018) An-aided transmit beamforming design for secured cognitive radio networks with swipt. Wirel Commun Mob Comput 2018
Zheng Z, Cui W, Qiao L, Guo J (2018) Performance and power consumption analysis of ieee802. 11ah for smart grid. Wirel Commun Mob Comput 2018
Wang X, Vasilakos AV, Chen M, Liu Y, Kwon TT (2012) A survey of green mobile networks: Opportunities and challenges. Mob Netw Appl 17(1):4–20
Adelin A, Owezarski P, Gayraud T (2010) On the impact of monitoring router energy consumption for greening the internet. In: Grid computing (GRID), 11th IEEE/ACM international conference on. IEEE, Conference Proceedings, pp 298– 304
Yang Y, Wang D, Pan D, Xu M (2016) Wind blows, traffic flows: Green internet routing under renewable energy. In: Computer communications, IEEE INFOCOM-The 35th Annual IEEE international conference on. IEEE, Conference Proceedings, pp 1–9
Hoque MA, Siekkinen M, Nurminen JK (2014) Energy efficient multimedia streaming to mobile devices—a survey. IEEE Commun Surv Tutor 16(1):579–597
Al Ridhawi I, Otoum S, Aloqaily M, Jararweh Y, Baker T (2020) Providing secure and reliable communication for next generation networks in smart cities. Sustainable Cities and Society 56:102080
Lloret J, Garcia M, Bri D, Sendra S (2009) A wireless sensor network deployment for rural and forest fire detection and verification. Sensors 9(11):8722–8747
Aslan YE, Korpeoglu I, Ulusoy Z (2012) A framework for use of wireless sensor networks in forest fire detection and monitoring Computers. Environ Urban Syst 36(6):614–625
Bhattacharjee S, Roy P, Ghosh S, Misra S, Obaidat MS (2012) Wireless sensor network-based fire detection, alarming, monitoring and prevention system for bord-and-pillar coal mines. J Syst Softw 85(3):571–581
Viani F, Lizzi L, Rocca P, Benedetti M, Donelli M, Massa A (2008) Object tracking through rssi measurements in wireless sensor networks. Electron Lett 44(10):653–654
Han G, Shen J, Liu L, Qian A, Shu L (2016) Tgm-cot: energy-efficient continuous object tracking scheme with two-layer grid model in wireless sensor networks. Pers Ubiquit Comput 20(3):349–359
Han G, Shen J, Liu L, Shu L (2017) Brtco: A novel boundary recognition and tracking algorithm for continuous objects in wireless sensor networks. IEEE Systems Journal
Wu F, Rüdiger C, Yuce MR (2017) Real-time performance of a self-powered environmental iot sensor network system. Sensors 17(2):282
Prabhu B, Balakumar N, Antony AJ (2017) Wireless sensor network based smart environment applications
Trasviña-Moreno CA, Blasco R, Marco L, Casas R, Trasviña-Castro A (2017) Unmanned aerial vehicle based wireless sensor network for marine-coastal environment monitoring. Sensors 17(3):460
Sharma D (2017) Low cost experimental set up for real time temperature, humidity monitoring through wsn. Int J Eng Sci 4340
Almalki SHA, Faris A, Othman SB, Sakli H (2021) A low-cost platform for environmental smart farming monitoring system based on iot and uavs. Sustainability
Prabhu B, Balakumar N, Antony AJ (2017) Evolving constraints in military applications using wireless sensor networks
Ye W, Heidemann J, Estrin D (2002) An energy-efficient mac protocol for wireless sensor networks. In: INFOCOM Twenty-first annual joint conference of the IEEE computer and communications societies. Proceedings IEEE, vol 3. IEEE, Conference Proceedings, pp 1567–1576
Anastasi G, Francesco MD, Conti M, Passarella A (2013) How to prolong the lifetime of WSNs. CRC Press, Boca Raton. book Section 6
Khalil HB, Zaidi SJH (2012) Mnmu-ra: Most nearest most used routing algorithm for greening the wireless sensor networks. Wirel Sens Netw 4(06):162
Azevedo J, Santos F (2012) Energy harvesting from wind and water for autonomous wireless sensor nodes. IET Circ Dev Syst 6(6):413–420
Article MathSciNet Google Scholar
Eu ZA, Tan H-P, Seah WK (2011) Design and performance analysis of mac schemes for wireless sensor networks powered by ambient energy harvesting. Ad Hoc Netw 9(3):300–323
Shaikh FK, Zeadally S (2016) Energy harvesting in wireless sensor networks: a comprehensive review. Renew Sust Energ Rev 55:1041–1054
Hawbani A, Wang X, Al-Dubai A, Zhao L, Busaileh O, Liu P, Al-qaness MAA (2021) A novel heuristic data routing for urban vehicular ad-hoc networks. IEEE Internet of Things Journal
Busaileh O, Hawbani A, Wang X, Liu P, Zhao L, Al-Dubai AY (2020) Tuft: Tree based heuristic data dissemination for mobile sink wireless sensor networks. IEEE Transactions on Mobile Computing
Hawbani A, Wang X, Zhao L, Al-Dubai A, Min G, Busaileh O (2020) Novel architecture and heuristic algorithms for software-defined wireless sensor networks. IEEE/ACM Trans Netw 28 (6):2809–2822
Jain PC (2015) Recent trends in energy harvesting for green wireless sensor networks. In: International conference on signal processing and communication (ICSC) conference proceedings, pp 40–45
Abedin SF, Alam MGR, Haw R, Hong CS (2015) A system model for energy efficient green-iot network. In: Information networking (ICOIN) international conference on. IEEE, Conference Proceedings, pp 177–182
Sun K, Ryoo I (2015) A study on medium access control scheme for energy efficiency in wireless smart sensor networks. In: Information and communication technology convergence (ICTC) international conference on. IEEE, Conference Proceedings, pp 623–625
Uzoh PC, Li J, Cao Z, Kim J, Nadeem A, Han K (2015) Energy efficient sleep scheduling for wireless sensor networks. In: International conference on algorithms and architectures for parallel processing. Springer, Conference Proceedings, pp 430–444
Mehmood A, Song H (2015) Smart energy efficient hierarchical data gathering protocols for wireless sensor networks. SmartCR 5(5):425–462
Rekha RV, Sekar JR (2016) An unified deployment framework for realization of green internet of things (giot). Middle-East J Sci Res 24(2):187–196
Naranjo PGV, Shojafar M, Mostafaei H, Pooranian Z, Baccarelli E (2017) P-sep: A prolong stable election routing algorithm for energy-limited heterogeneous fog-supported wireless sensor networks. J Supercomput 73(2):733–755
Yaacoub E, Kadri A, Abu-Dayya A (2012) Cooperative wireless sensor networks for green internet of things. In: Proceedings of the 8h ACM symposium on QoS and security for wireless and mobile networks. ACM, Conference Proceedings, pp 79– 80
Castillo-Cara M, Huaranga-Junco E, Quispe-Montesinos M, Orozco-Barbosa L, Antúnez EA (2018) Frog: a robust and green wireless sensor node for fog computing platforms. J Sensors 2018
Mahapatra C, Sheng Z, Kamalinejad P, Leung VC, Mirabbasi S (2017) Optimal power control in green wireless sensor networks with wireless energy harvesting, wake-up radio and transmission control. IEEE Access 5:501–518
Amirthavarshini LJ, Varshini R , Kavya S (2015) Wireless Sensor Networks in Green Cloud Computing. International Journal of Scientific & Engineering Research 6(10):98–100. https://www.ijser.org/researchpaper/Wireless-Sensor-Networks-in-Green-Cloud-Computing.pdf https://www.ijser.org/researchpaper/Wireless-Sensor-Networks-in-Green-Cloud-Computing.pdf
Khatri A, Kumar S, Kaiwartya O, Aslam N, Meena N, Abdullah AH (2018) Towards green computing in wireless sensor networks: Controlled mobility–aided balanced tree approach. Int J Commun Syst 31(7):e3463
Araujo A, Romero E, Blesa J, Nieto-Taladriz O (2012) Cognitive wireless sensor networks framework for green communications design. In: Proceedings of the 2nd international conference on advances in cognitive radio (COCORA’12), conference proceedings, pp 34–40
Rault T, Bouabdallah A, Challal Y (2014) Energy efficiency in wireless sensor networks: a top-down survey. Comput Netw 67:104–122
Seuwou P, Banissi E, Ubakanma G (2020) The future of mobility with connected and autonomous vehicles in smart cities. Springer, Berlin, pp 37–52
Bencardino M, Greco I (2014) Smart communities. social innovation at the service of the smart cities, Tema. Journal of Land Use, Mobility and Environment
Jraisat L (2020) Information sharing in sustainable value chain network (SVCN)—-the perspective of transportation in Cities. Springer, Berlin, pp 67–77
Yoo S-J, Park J-H, Kim S-H, Shrestha A (2016) Flying path optimization in uav-assisted iot sensor networks. ICT Express 2(3):140–144
Mozaffari M, Saad W, Bennis M, Debbah M (2015) Drone small cells in the clouds: Design, deployment and performance analysis. In: Global communications conference (GLOBECOM), IEEE. IEEE, Conference Proceedings, pp 1–6
Cao H-R, Yang Z, Yue X-J, Liu Y-X (2017) An optimization method to improve the performance of unmanned aerial vehicle wireless sensor networks. Int J Distrib Sensor Netw 13(4):1550147717705614
Cao H, Liu Y, Yue X, Zhu W (2017) Cloud-assisted uav data collection for multiple emerging events in distributed wsns. Sensors 17(8):1818
Dong M, Ota K, Lin M, Tang Z, Du S, Zhu H (2014) Uav-assisted data gathering in wireless sensor networks. J Supercomput 70(3):1142–1155
Zorbas D, Razafindralambo T, Guerriero F (2013) Energy efficient mobile target tracking using flying drones. Procedia Comput Sci 19:80–87
Sharma V, You I, Kumar R (2016) Energy efficient data dissemination in multi-uav coordinated wireless sensor networks. Mob Inform Syst 2016
Uragun B (2011) Energy efficiency for unmanned aerial vehicles. In: Machine learning and applications and workshops (ICMLA), 10th international conference on, vol 2. IEEE, Conference Proceedings, pp 316–320
Choi DH, Kim SH, Sung DK (2014) Energy-efficient maneuvering and communication of a single uav-based relay. IEEE Trans Aerosp Electron Syst 50(3):2320–2327
Yu Y, Lee S, Lee J, Cho K, Park S (2016) Design and implementation of wired drone docking system for cost-effective security system in iot environment. In: Consumer electronics (ICCE) IEEE international conference on. IEEE, Conference Proceedings, pp 369–370
Seo S-H, Choi J-I, Song J (2017) Secure utilization of beacons and uavs in emergency response systems for building fire hazard. Sensors 17(10):2200
Fujii K, Higuchi K, Rekimoto J (2013) Endless flyer: a continuous flying drone with automatic battery replacement. In: Ubiquitous intelligence and computing, IEEE 10th international conference on and 10th international conference on autonomic and trusted computing (UIC/ATC). IEEE, Conference Proceedings, pp 216–223
Sahal R (2021) Digital twins collaboration for automatic erratic operational data detection in industry 4.0. Appl Sci 11:15
Luo Z, Zhong L, Zhang Y, Miao Y, Ding T (2017) An efficient intelligent algorithm based on wsns of the drug control system. Tehnički vjesnik 24(1):273–282
Mahapatra C, Moharana AK, Leung V (2017) Energy management in smart cities based on internet of things: Peak demand reduction and energy savings. Sensors 17(12):2812
Rani S, Chauhdary SH (2018) A novel framework and enhanced qos big data protocol for smart city applications
Shafik W, Matinkhah SM, Ghasemzadeh M (2020) Internet of things-based energy management, challenges, and solutions in smart cities. J Commun Technol Electron Comput Sci 27:1–11
Villa TF, Salimi F, Morton K, Morawska L, Gonzalez F (2016) Development and validation of a uav based system for air pollution measurements. Sensors 16(12):2202
Wang J, Schluntz E, Otis B, Deyle T (2015) A new vision for smart objects and the internet of things: Mobile robots and long-range uhf rfid sensor tags, arXiv: 1507.02373
Hamilton A, Magdalene AHS (2017) Study of solar powered unmanned aerial vehicle to detect greenhouse gases by using wireless sensor network technology. J Sci Eng Educ (ISSN 2455-5061) 2:1–11
Almalki FA (2020) Utilizing drone for food quality and safety detection using wireless sensors. In: IEEE 3rd international conference on information communication and signal processing (ICICSP). IEEE, Conference Proceedings, pp 405–412
Klimkowska A, Lee I, Choi K (2016) Possibilities of uas for maritime monitoring, ISPRS-international Archives of the Photogrammetry. Remote Sens Spatial Inform Sci 885–891
Villa TF, Gonzalez F, Miljievic B, Ristovski ZD, Morawska L (2016) An overview of small unmanned aerial vehicles for air quality measurements: Present applications and future prospectives. Sensors 16 (7):1072
Telesetsky A (2016) Navigating the legal landscape for environmental monitoring by unarmed aerial vehicles. Geo Wash J Energy Envtl L 7:140
Alvear O, Calafate CT, Hernández E, Cano J-C, Manzoni P (2015) Mobile pollution data sensing using uavs. In: Proceedings of the 13th international conference on advances in mobile computing and multimedia. ACM, Conference Proceedings, pp 393–397
Alvear OA, Zema NR, Natalizio E, Calafate CT (2017) A chemotactic pollution-homing uav guidance system. In: Wireless communications and mobile computing conference (IWCMC), 13th International. IEEE, Conference Proceedings, pp 2115–2120
Alvear O, Zema NR, Natalizio E, Calafate CT (2017) Using uav-based systems to monitor air pollution in areas with poor accessibility. J Adv Transport 2017
Koo VC, Chan YK, Vetharatnam G, Chua MY, Lim CH, Lim C-S, Thum C, Lim TS, bin Ahmad Z, Mahmood KA (2012) A new unmanned aerial vehicle synthetic aperture radar for environmental monitoring. Prog Electromagn Res 122:245– 268
Šmídl V, Hofman R (2013) Tracking of atmospheric release of pollution using unmanned aerial vehicles. Atmos Environ 67:425–436
Zang W, Lin J, Wang Y, Tao H (2012) Investigating small-scale water pollution with uav remote sensing technology. In: World automation congress (WAC). IEEE, Conference Proceedings, pp 1–4
Bronk C, Lingamneni A, Palem K (2010) Innovation for sustainability in information and communication technologies (ict). In: James A Baker III Institute for Public Policy Rice University
Gutierrez JM, Jensen M, Henius M, Riaz T (2015) Smart waste collection system based on location intelligence. Procedia Comput Sci 61:120–127
Omar M, Termizi A, Zainal D, Wahap N, Ismail N, Ahmad N (2016) Implementation of spatial smart waste management system in malaysia. In: IOP conference series: Earth and environmental science, vol 37. IOP Publishing, Conference Proceedings, p 012059
Popescu DE, Bungau C, Prada M, Domuta C, Bungau S, Tit D (2016) Waste management strategy at a public university in smart city context. J Environ Prot Ecol 17(3):1011–1020
Del Borghi A, Gallo M, Strazza C, Magrassi F, Castagna M (2014) Waste management in smart cities: The application of circular economy in genoa (italy). Impresa Progetto Electronic Journal of Management 4:1–13
Vu DD, Kaddoum G (2017) A waste city management system for smart cities applications. In: Advances in wireless and optical communications (RTUWO). IEEE, Conference Proceedings, pp 225–229
Shyam GK, Manvi SS, Bharti P (2017) Smart waste management using internet-of-things (iot). In: Computing and communications technologies (ICCCT), 2nd International Conference on. IEEE, Conference Proceedings, pp 199–203
Aazam M, St-Hilaire M, Lung C-H, Lambadaris I (2016) Cloud-based smart waste management for smart cities. In: Computer aided modelling and design of communication links and networks (CAMAD) IEEE 21st international workshop on. IEEE, Conference Proceedings, pp 188–193
Sivasankari A, Priyavadana V (2016) Smart planning in solid waste management for a sustainable smart city. Int Res J Eng Technol 3(8):2051–2061
Popa CL, Carutasu G, Cotet CE, Carutasu NL, Dobrescu T (2017) Smart city platform development for an automated waste collection system. Sustainability 9(11):2064
Pirlone F, Spadaro I (2014) Towards a waste management plan for smart cities. WIT Trans Ecol Environ 191:1279–1290
Ismagiloiva E, Hughes L, Rana N, Dwivedi Y (2019) Role of smart cities in creating sustainable cities and communities: A systematic literature review. In: International working conference on transfer and diffusion of IT. Springer, Conference Proceedings, pp 311–324
Maksimovic M (2017) The role of green internet of things (g-iot) and big data in making cities smarter, safer and more sustainable. Int J Comput Digit Syst 6(04):175–184
Sodhro AH, Pirbhulal S, Luo Z, de Albuquerque VHC (2019) Towards an optimal resource management for iot based green and sustainable smart cities. J Clean Prod 220:1167–1179
Tuysuz MF, Trestian R (2020) From serendipity to sustainable green iot: technical, industrial and political perspective. Comput Netw 182:107469
Maksimovic M (2018) Greening the future: Green Internet of Things (G-IoT) as a key technological enabler of sustainable development. Springer, Berlin, pp 283–313
Kumar A, Payal M, Dixit P, Chatterjee JM (2020) Framework for realization of green smart cities through the internet of things (iot). Trends in Cloud-based IoT 85–111
Sharma SK, Gayathri N, Kumar SR, Ramesh C, Kumar A, Modanval RK (2021) Green ICT, Communication, networking, and data processing. Springer, Berlin, pp 151–170
Dell’Anna F (2021) Green jobs and Energy efficiency as strategies for economic growth and the reduction of environmental impacts. Energ Policy 149:112031
Chithaluru P, Al-Turjman F, Kumar M, Stephan T (2020) I-areor: An energy-balanced clustering protocol for implementing green iot in smart cities. Sustain Cities Soc 61:102254
Cetin C, Karafakı FC (2020) The influence of green areas on city-dwellers’ perceptions of air pollution The case of nigde city center. J Environ Biol 41(2):453–461
Mingaleva Z, Vukovic N, Volkova I, Salimova T (2020) Waste management in green and smart cities: a case study of russia. Sustainability 12(1):94
Ali T, Irfan M, Alwadie AS, Glowacz A (2020) Iot-based smart waste bin monitoring and municipal solid waste management system for smart cities. Arab J Sci Eng 45:10185–10198
Elayyan HO (2021) Sustainability and smart cities: a case study of internet radio. Springer, Berlin, pp 281–296
Ortega-Fernández A, Martín-Rojas R, García-Morales VJ (2020) Artificial intelligence in the urban environment: Smart cities as models for developing innovation and sustainability. Sustainability 12 (19):7860
Alsamhi SH, Almalki FA, Ma O, Ansari MS, Angelides MC (2019) Performance optimization of tethered balloon technology for public safety and emergency communications. Telecommun Syst 1–10
Alsamhi SH, Ansari MS, Ma O, Almalki F, Gupta SK (2019) Tethered balloon technology in design solutions for rescue and relief team emergency communication services. Disaster Medicine and Public Health Preparedness 13(2):203– 210
Alsamhi SH, Ansari MS, Rajput NS (2018) Disaster coverage predication for the emerging tethered balloon technology: capability for preparedness, detection, mitigation, and response. Disaster Medicine and Public Health Preparedness 12(2):222–231
Download references
Acknowledgements
This research has emanated from research supported by a research grant from Science Foundation Ireland (SFI) under Grant Number SFI/16/RC/3918 (Confirm), and Marie Skłodowska-Curie grant agreement No. 847577 co-funded by the European Regional Development Fund.
The authors are grateful to the Deanship of Scientific Research at Taif University, Kingdom ofSaudi Arabia for funding this project through Taif University ResearchersSupporting Project Number (TURSP-2020/265).
Author information
Authors and affiliations.
Department of computer engineering, collage of computers and information technology, Taif University, Taif, Kingdom of Saudi Arabia
Faris. A. Almalki
Athlone Institute of Technology, Athlone, Ireland
S. H. Alsamhi
IBB University, Ibb, Yemen
SMART 4.0 Fellow, CONFIRM Centre for Smart Manufacturing, University College, Cork, Ireland
Radhya Sahal
Faculty of Computer Science and Engineering, Hodeidah University, Al Hodeidah, Yemen
School of Engineering and Technology, CQUniversity Australia, Rockhampton, Australia
Jahan Hassan
School of Computer Science and Technology, University of Science and Technology of China, Hefei, China
Ammar Hawbani
Department of Electronics Engineering, IIT (BHU), Varanasi, India
N. S. Rajput
Department of Electrical Engineering, Faculty of Engineering, University of Malaya, 50603, Kuala Lumpur, Malaysia
National University of Ireland Galway, Galway, Ireland
Jeff Morgan & John Breslin
You can also search for this author in PubMed Google Scholar
Corresponding author
Correspondence to S. H. Alsamhi .
Additional information
Publisher’s note.
Springer Nature remains neutral with regard to jurisdictional claims in published maps and institutional affiliations.
Rights and permissions
Open Access This article is licensed under a Creative Commons Attribution 4.0 International License, which permits use, sharing, adaptation, distribution and reproduction in any medium or format, as long as you give appropriate credit to the original author(s) and the source, provide a link to the Creative Commons licence, and indicate if changes were made. The images or other third party material in this article are included in the article's Creative Commons licence, unless indicated otherwise in a credit line to the material. If material is not included in the article's Creative Commons licence and your intended use is not permitted by statutory regulation or exceeds the permitted use, you will need to obtain permission directly from the copyright holder. To view a copy of this licence, visit http://creativecommons.org/licenses/by/4.0/ .
Reprints and permissions
About this article
Almalki, F.A., Alsamhi, S.H., Sahal, R. et al. Green IoT for Eco-Friendly and Sustainable Smart Cities: Future Directions and Opportunities. Mobile Netw Appl 28 , 178–202 (2023). https://doi.org/10.1007/s11036-021-01790-w
Download citation
Accepted : 20 May 2021
Published : 17 August 2021
Issue Date : February 2023
DOI : https://doi.org/10.1007/s11036-021-01790-w
Share this article
Anyone you share the following link with will be able to read this content:
Sorry, a shareable link is not currently available for this article.
Provided by the Springer Nature SharedIt content-sharing initiative
- Sustainability
- Eco-friendly
- Energy efficiency
- Find a journal
- Publish with us
- Track your research
- Research Article
- Open access
- Published: 28 August 2019
Generating a vision for smart sustainable cities of the future: a scholarly backcasting approach
- Simon Elias Bibri 1 , 2 &
- John Krogstie 1
European Journal of Futures Research volume 7 , Article number: 5 ( 2019 ) Cite this article
25k Accesses
56 Citations
Metrics details
Sustainable cities have been the leading global paradigm of urbanism. Undoubtedly, sustainable development has, since its widespread diffusion in the early 1990s, positively influenced city planning and development. This pertains to the immense opportunities that have been explored and the enormous benefits that have been realized in relation to sustainable urban forms, especially compact cities and eco-cities. However, such forms are still associated with a number of problems, issues, and challenges. This mainly involves the question of how they should be monitored, understood, analyzed, and planned to improve, advance, and maintain their contribution to sustainability and thus to overcome the kind of wicked problems, unsettled issues, and complex challenges they embody. This in turn brings us to the current question related to the weak connection between and the extreme fragmentation of sustainable cities and smart cities as approaches and landscapes, respectively, despite the proven role of advanced ICT, coupled with the untapped potential of big data technology and its novel applications, in supporting sustainable cities as to enhancing and optimizing their performance under what is labeled “smart sustainable cities.” In this respect, there has recently been a conscious push for sustainable cities to become smart and thus more sustainable by particularly embracing what big data technology and its novel applications has to offer in the hopes of reaching the optimal level of sustainability. In the meantime, we are in the midst of an expansion of time horizons in city planning and development. In this context, sustainable cities across the globe have adopted ambitious smart goals that extend far into the future. Essentially, there are multiple visions of, and pathways to achieving, smart sustainable cities based on how they can be conceptualized and operationalized. The aim of this paper is to generate a vision for smart sustainable cities of the future by answering the 6 guiding questions for step 3 of the futures study being conducted. This study aims to analyze, investigate, and develop a novel model for smart sustainable cities of the future using backcasting as a scholarly approach. It involves a series of papers of which this paper is the second one, following the earlier papers with steps 1 and 2. Visionary images of a long-term future can stimulate an accelerated movement towards achieving the long-term goals of sustainability. The proposed model is believed to be the first of its kind and thus has not been, to the best of one’s knowledge, produced, nor is it being currently investigated, elsewhere.
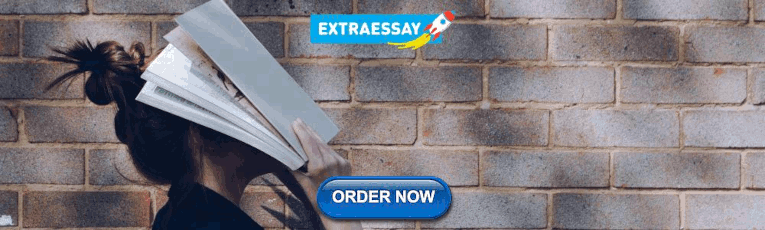
Introduction
Contemporary cities have a pivotal role in strategic sustainable development; therefore, they have gained a central position in operationalizing this notion and applying this discourse. This is clearly reflected in the Sustainable Development Goal 11 (SGD 11) of the United Nations’ 2030 Agenda, which seek to make cities more sustainable, resilient, inclusive, and safe [ 73 ]. Sustainable cities have long been the leading global paradigm of urbanism [ 19 , 74 , 77 , 78 , 79 ] for more than three decades or so. The subject of “sustainable cities” remains endlessly fascinating and enticing, as there are numerous actors involved in the academic and practical aspects of the endeavor, including engineers and architects, green technologists, built and natural environment specialists, and environmental and social scientists, and, more recently, computer scientists, data scientists, and urban scientists. All these actors are undertaking research and developing strategies and programs to tackle the challenging elements of sustainable urbanism. This adds to the work of policymakers and political decision-makers in terms of formulating and implementing regulatory policies and devising and applying political mechanisms and governance arrangements to promote and spur innovation and monitor and maintain progress in sustainable cities.
Since its widespread diffusion in the early 1990s, sustainable development has had significant positive impacts on the planning and development of cities in terms of the different dimensions of sustainability. It has also revived the discussion about the form of cities [ 40 ]. In this regard, it has inspired a whole generation of urban scholars and practitioners into a quest for the immense opportunities and fascinating possibilities that could be enabled and created by, and the enormous benefits that could be realized from, the planning and development of sustainable urban forms (especially compact cities and eco-cities), That is to say, forms for human settlements that can meet the required level of sustainability and enable the built environment to function in a constructive way. This can be accomplished through continuously improving their contribution to the goals of sustainable development in terms of reducing material use, lowering energy consumption, mitigating pollution, and minimizing waste, as well as in terms of improving equity, inclusion, the quality of life, and well-being.
However, new circumstances require new responses. This pertains to the spread of urbanization and the rise of ICT and how they are drastically changing sustainable urbanism. The transformative force of urbanization and ICT and the role that cities can play have far-reaching implications. By all indicators, the urban world will become largely technologized and computerized within just a few decades, and ICT as an enabling, integrative, and constitutive technology of the twenty-first century will accordingly be instrumental, if not determining, in addressing many of the conundrums posed, the issues raised, and the challenges presented by urbanization. It is therefore of strategic value to start directing the use of emerging ICT into understanding and proactively mitigating the potential effects of urbanization, with the primary aim of tackling the many intractable and wicked problems involved in urban operational functioning, management, planning, development, and governance, especially in the context of sustainability. Indeed, the rapid urbanization of the world poses significant and unprecedented challenges associated with sustainability (e.g., [ 26 , 31 , 34 ]) due to the issues engendered by urban growth. In short, the multidimensional effects of unsustainability are most likely to exacerbate with urbanization. Urban growth will jeopardize the sustainability of cities [ 53 ]. Therefore, ICT has come to the fore and become of crucial importance for containing the effects of urbanization and facing the challenges of sustainability, including in the context of sustainable cities which are striving to improve, advance, and maintain their contribution to the goals of sustainable development. The use of advanced ICT in sustainable cities constitutes an effective approach to decoupling the health of the city and the quality of life of citizens from the energy and material consumption and concomitant environmental risks associated with urban operations, functions, services, strategies, and policies [ 13 ].
Smart sustainable cities as an integrated and holistic approach to urbanism represent an instance of sustainable urban planning and development, a strategic approach to achieving the long-term goals of urban sustainability—with support of advanced technologies and their novel applications. Accordingly, achieving the status of smart sustainable cities epitomizes an instance of urban sustainability. This notion refers to a desired (normative) state in which a city strives to retain a balance of the socio-ecological systems through adopting and executing sustainable development strategies as a desired (normative) trajectory [ 19 ]. This balance entails enhancing the physical, environmental, social, and economic systems of the city in line with sustainability over the long run—given their interdependence, synergy, and equal importance. This long-term strategic goal requires, as noted by [ 7 ], p. 601), “fostering linkages between scientific research, technological innovations, institutional practices, and policy design and planning in relevance to sustainability. It also requires a long-term vision, a trans-disciplinary approach, and a system-oriented perspective on addressing environmental, economic, social, and physical issues.” All these requirements are at the core of backcasting as a scholarly and planning approach to futures studies. This approach facilitates and contributes to the development, implementation, evaluation, and improvement of models for smart sustainable cities, with a particular focus on practical interventions for integrating and improving urban systems and coordinating and coupling urban domains using cutting-edge technologies in line with the vision of sustainability. One of the most appealing strands of research in the domain of smart sustainable urbanism is that which is concerned with futures studies. The relevance and rationale behind futures research approach are linked to the strategic planning and development associated with long-term sustainability endeavors, initiatives, or solutions. And backcasting is well suited to any multifaceted kind of planning and development process (e.g., [ 38 ]), as well as to dealing with urban sustainability problems and challenges [ 19 , 23 , 29 , 52 , 59 ].
The aim of this paper is to generate a vision for smart sustainable cities of the future by answering the 6 guiding questions for step 3 of the futures study being conducted, namely:
What are the terms of reference for the future vision?
How does the future sustainable socio-technical system and need fulfillment look like?
How is the future vision different from the existing socio-technical systems?
What is the rationale for developing the future vision?
Which sustainability problems, issues, and challenges have been dealt with by meeting the stated objectives and thus achieving the specified goals?
Which advanced technologies and their novel applications have been used in the future vision?
This futures study aims to analyze, investigate, and develop a novel model for smart sustainable cities of the future using backcasting as a scholarly approach. It consists of 6 steps in total and a number of guiding questions for each step to answer. Accordingly, it involves a series of papers of which this paper is the second one, following the earlier papers with steps 1 and 2: strategic problem orientation [ 19 ]. This paper as a sequel leads through the whole of the backcasting study: step 4 with 2 papers, step 5 with 1 paper, and step 6 with 1 paper. All in all, as this is an extensive scholarly project involving description, investigation, synthesis, design, analysis, and compilation, it is deemed more appropriate to divide it into a series of papers.
The remainder of this paper is structured as follows. Section 2 provides a background of the futures study, including a review of the area being researched, the issue of the current situation, and studies and relevant history on the issue. Section 3 focuses on the backcasting methodology, with an emphasis on step 3. Section 4 delves into step 3 of the futures study by answering the 6 guiding questions in more detail following the applied backcasting approach. This paper ends, in Section 5 , with discussion and conclusion.
Background of the futures study
Sustainable cities are associated with a number of problems, issues, and challenges (i.e., deficiencies, Limitations difficulties, fallacies, and uncertainties) when it comes to their management, planning, design, development, and governance in the context of sustainability (e.g., [ 16 , 17 , 19 , 27 , 28 , 54 ]). This mainly involves the question of how sustainable urban forms should be monitored, understood, and analyzed in order to be effectively planned, designed, developed, managed, and governed in terms of enhancing and maintaining their sustainability performance [ 13 ]. The underlying argument is that more innovative solutions and sophisticated approaches are needed to overcome the kind of wicked problems, unsettled issues, and complex challenges pertaining to sustainable urban forms. This brings us to the current question related to the weak connection of and extreme fragmentation between sustainable cities and smart cities as approaches and landscapes, respectively (e.g., [ 3 , 7 , 13 , 16 , 19 , 20 , 49 ]), despite the great potential of advanced ICT for, and its proven role in, supporting sustainable cities in improving their performance under what is labeled “smart sustainable cities” (e.g., see, [ 7 , 8 , 17 , 49 , 68 ]). In particular, tremendous opportunities are available for utilizing big data computing and the underpinning technologies and their novel applications in sustainable cities to improve, advance, and maintain their contribution to the goals of sustainable development. The main strength of the big data technology is the high influence it will have on many facets of smart sustainable cities and their citizens’ lives (see, e.g., [ 2 , 3 , 4 , 6 , 8 , 13 , 58 , 71 ]).
In light of the above, recent research endeavors have started to focus on smartening up sustainable cities through enhancing and optimizing their operational functioning, management, planning, design, development, and governance in line with the long-term vision of sustainability under what is labeled “smart sustainable cities”([ 7 , 8 , 9 , 12 , 16 , 17 , 19 ], Bibri and Krogstie 2017c). This wave of research revolves around integrating the landscapes of, and the approaches to, sustainable cities and smart cities in a variety of ways in the hopes of reaching the required level of sustainability and improving the living standard of citizens [ 13 ]. This integrated approach tends to take several forms in terms of combining the strengths of sustainable cities and smart cities based on how the concept of smart sustainable cities can be conceptualized and operationalized, just as it has been the case for sustainable cities. Indeed, several topical studies (e.g., [ 3 , 7 , 8 , 13 , 17 , 49 , 50 , 62 , 68 , 81 ]) have addressed the merger of the sustainable city and smart city approaches from a variety of perspectives. Accordingly, there is a host of opportunities yet to explore towards new approaches to smart sustainable urbanism. The focus in this paper is on integrating the design principles and planning practices of sustainable urban forms with big data computing and the underpinning technologies and their novel applications being offered by smart cities of the future. The underlying assumption is that the evolving big data deluge with its extensive sources hides in itself the answers to the most challenging analytical questions as well as the solutions to the most complex challenges pertaining to sustainability in the face of urbanization. It also plays a key role in understanding urban constituents as data agents.
In recent years, there has been a marked intensification of datafication. This is manifested in a radical expansion in the volume, range, variety, and granularity of the data being generated about urban environments and citizens (e.g., [ 46 , 47 , 48 ]), with the primary aim of quantifying the whole of the city, putting it in a data format so it can be organized and analyzed [ 13 ]. We are currently experiencing the accelerated datafication of the city in a rapidly urbanizing world and witnessing the dawn of the big data era not out of the window, but in everyday life. Our urban everydayness is entangled with data sensing, data processing, and communication networking, and our wired world generates and analyzes overwhelming and incredible amounts of data. The modern city is turning into constellations of instruments and computers across many scales and morphing into a haze of software instructions, which are becoming essential to the operational functioning, planning, design, development, and governance of the city. The datafication of spatiotemporal citywide events has become a salient factor for the practice of smart sustainable urbanism.
As a consequence of datafication, a new era is presently unfolding wherein smart sustainable urbanism is increasingly becoming data-driven. At the heart of such urbanism is a computational understanding of urban systems and processes that renders urban life a form of logical rules and algorithmic procedures—which is underpinned and informed by data-intensive scientific approaches to urban science and urban sustainability, while also harnessing urban big data to provide a more holistic and integrated view and synoptic intelligence of the city [ 13 ]. This is increasingly directed towards improving, advancing, and maintaining the contribution of sustainable cities to the goals of sustainable development in an increasingly urbanized world.
We are living at the dawn of what has been termed as “the fourth paradigm of science,” a scientific revolution that is marked by the recent emergence of big data science and analytics as well as the increasing adoption and use of the underlying technologies in scientific and scholarly research practices. Everything about science development and knowledge production is fundamentally changing thanks to the unfolding and soaring data deluge. The upcoming data avalanche is thus the primary fuel of this new age, which powerful computational processes or analytics algorithms are using to generate useful knowledge and deep insights pertaining to a wide variety of practical uses.
As a new area of science and technology, “big data science and analytics embodies an unprecedentedly transformative power—which is manifested not only in the form of revolutionizing science and transforming knowledge, but also in advancing social practices, catalyzing major shifts, and fostering societal transitions. Of particular relevance, it is instigating a massive change in the way both sustainable cities and smart cities are understood, studied, planned, operated, and managed to improve and maintain sustainability in the face of expanding urbanization” ([ 14 ], p. 79). To put it differently, these practices are becoming highly responsive to a form of data-driven urbanism that is the key mode of production for what have been termed smart sustainable cities whose monitoring, understanding, and analysis are increasingly relying on big data technologies.
In a nutshell, the Fourth Scientific Revolution is set to erupt in cities, break out suddenly and dramatically, throughout the world. This is manifested in bits meeting bricks on a vast scale as instrumentation, datafication, and computerization are permeating the spaces we live in. The outcome will impact most aspects of urban life, raising questions and issues of urgent concern, especially those related to sustainability and urbanization. This pertains to what dimensions of cities will be most affected; how urban planning, design, development, and governance should change and evolve; and, most importantly, how cities can embrace and prepare for looming technological disruptions and opportunities.
In light of the above, at the beginning of a new decade, we have the opportunity to look forward and consider what we could achieve in the coming years in the era of big data revolution. Again, we have the chance to consider the desired future of data-driven smart sustainable cities. This will motivate many urban scholars, scientists, and practitioners to think about how the subject of “data-driven smart sustainable cities” might develop, as well as inspire them into a quest for the immense opportunities and fascinating possibilities that can be created by the development and implementation of such cities. In this respect, we are in the midst of an expansion of time horizons in city planning. Sustainable cities look further into the future when forming scenarios and strategies to achieve them. The movement towards a long-term vision arises from three major mega trends or macro-shifts that shape our societies at a growing pace: sustainability, ICT, and urbanization. Recognizing a link between such trends, sustainable cities across the globe have adopted ambitious goals that extend far into the future and developed different pathways to achieve them.
Backcasting as a scholarly approach to strategic smart sustainable city planning and development
As a special kind of scenario methodology, backcasting is applied here to build a future model for smart sustainable cities as a planning tool for facilitating urban sustainability. Backcasting scenarios are used to explore future uncertainties, create opportunities, build capabilities, and improve decision-making processes. Their primary aim is to discover alternative pathways through which a desirable future can be reached. Following Rotmans et al.’s [ 65 ] taxonomy, scenarios can be classified into different categories, including projective and prospective scenarios, qualitative and quantitative scenarios, participatory and expert scenarios, and descriptive and normative scenarios. This futures study is concerned with a normative scenario, which takes values and interests (sustainability and big data technology) into account and involves reasoning from specific long-term goals that have to be achieved.
In general, the backcasting approach is applicable in futures studies dealing with the fundamental question of backcasting, which involves the kind of actions that must be taken to achieve a long-term goal. In this context, if we want to attain a smart sustainable city, what actions must be taken to get there? Here backcasting means to look at the current situation from a future perspective. As an analytical and deliberative process (Fig. 1 ), backcasting entails articulating an end vision and then developing a pathway to get from the present to that endpoint. In more detail, the backcasting scenario is constructed from the distant future towards the present by defining a desirable future and then moving step-by-step backwards towards the present to identify the strategic steps that need to be taken to attain that specified future. This involves identifying the stumbling blocks on the way and the key stakeholders that should be involved to drive change, as well as developing and assessing the policy pathway in terms of planning practices and development strategies necessary to achieve the future outcome. The use of backcasting in futures studies assumes a vision of an evolutionary process of policy with a time frame of a generation or so, which is a basic principle to allow the policy actions to pursue the path towards, and potentially achieve, a sustainable future. Moreover, in urban sustainability, planning is about figuring out the ‘next steps’ which are quite literally the next concrete actions to undertake. Next steps are usually based on reacting to present circumstances, creativity, intuition, and common sense, but also (conceivably) are still aligned with the future vision and direction. Therefore, researchers in backcasting should not get obsessed with the next steps without considering how aligned they are with what they ultimately aim to achieve.
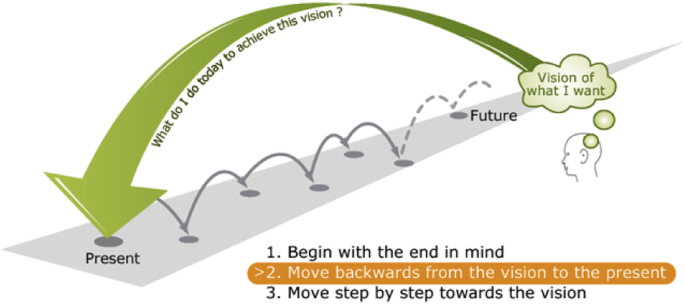
The backcasting process from the natural step. Source: Holmberg [ 37 ]
Figure 1 illustrates the backcasting process in which the future desired conditions are envisioned and steps are then defined to attain those conditions. In this regard, envisioning the smart sustainable city as a future vision has a normative side: what future is desired? Backcasting this preferred vision has an analytical side: how can this desirable future be attained? Backcasting is about analyzing possible ways of attaining certain futures as well as their feasibility and potential [ 56 ]. Specifically, in the quest for the answer to how to reach specified outcomes in the future, backcasting involves finding ways of linking goals that may lie far ahead in the future to a set of steps to be taken now and designed to achieve that end, and also facilitates discovery [ 29 ].
Backcasting is viewed as a natural step in operationalizing sustainable development [ 38 ] within different societal spheres. In terms of its practical application, backcasting is increasingly used in futures studies in the fields related to sustainable urban planning as a formal element of future strategic initiatives. It is the most applied approach in futures studies when it comes to sustainability problems and the identification and exploration of their solutions. This involves a wide variety of areas, including strategic city planning (e.g., [ 59 ]), sustainable city design [ 23 ]. transportation and mobility (Banister et al. 2000), sustainable transportation systems (Akerman and Höjer 2006; [ 39 , 66 ]), sustainable technologies and sustainable system innovation [ 76 ], sustainable household (Green and Vergragt 2002; [ 57 ]), and sustainable transformation of organizations [ 37 ]. Backcasting studies must reflect solutions to a specified social problem in the broader sense [ 29 ]. Bibri [ 10 ] concludes that the backcasting approach is found to be well-suited for long-term urban sustainability problems and solutions due to its normative, goal-oriented, and problem-solving character. Generally, as argued by Dreborg [ 29 ], backcasting is particularly useful when:
The problem to be studied is complex and there is a need for major change
The dominant trends are part of the problem
The problem to a great extent is a matter of externalities
The scope is wide enough and time horizon is long enough to leave considerable room for deliberate and different choices and directions of development.
Bibri [ 10 ] has recently conducted a comprehensive study on futures studies and related approaches. Its main focus is on backcasting as a scholarly and planning approach to strategic smart sustainable city development. Its main objectives are to review the existing backcasting methodologies and to discuss the relevance of their use in terms of their steps and guiding questions for analyzing, investigating, and developing smart sustainable cities, as well as to synthesize a backcasting approach based on a number of notable future studies. Later, Bibri and Krogstie [ 19 ] adapted the approach, i.e., made minor changes so as to improve and clarify it in accordance with the overall aim of this futures study as well as the specificity of the proposed model. Indeed, a commonly held view is that the researchers’ worldview and purpose remain the most important criteria for determining how futures studies can be developed and conducted in terms of the details concerning the questions guiding the steps involved in a particular backcasting approach. This helps to identify and implement strategic decisions associated with urban sustainability. However, the outcome of the adapted synthesized approach is illustrated in Table 1 .
As the focus in this paper is on step 3, it is important to point out that the backcasting approach is traditionally based on one normative vision, but multiple visions can also be used to explore different future alternatives (e.g., [ 72 ]). In this futures study, step 3 of backcasting constructs only one future vision based on the objectives, goals, and targets specified in step 1, indicating an integrated solution to a set of problems and challenges associated with existing sustainable urban forms, with support of advanced technologies. In addition, the development of the future vision is typically performed after the stage of analyzing the current situation and assessing the external factors (steps 1 and 2 of the backcasting study). While some views defend that a prior evaluation grounds the vision in realism, others argue that it curtails the ability to think of “ideal states” by putting the current circumstances and capabilities at the center of attention. However, this prescribed vision of the future is based on a sequential progression into the future of the current trends and the expected developments and the way they intertwine with and affect one another in relation to smart sustainable cities, without sharp transformation. It is also based on a combination of technological innovations and sustainability advancements, or on the co-evolutionary pathways of social and ecological systems.
Future vision generation
Constructing the future vision entails defining and describing a desirable future in which the problems and issues identified in relation to existing sustainable urban forms have been solved by meeting the stated objectives and thus achieving the specified goals and targets described in step 1 (see [ 19 ] for a detailed account and discussion). In general, future vision construction is about identifying the desired future state, which consists of vibrant descriptions of audacious goals and targets, as well as reflective statements addressing the aspired future. It is important to note at this stage that the vision of the future and the proposed novel model tend to be used interchangeably in this paper. Indeed, this vision represents a short and concise version of this model. In other words, this model entails a desired future state that is supposed to be more detailed at the end of this scholarly backcasting endeavor.
On the visionary approach (see guiding question 1)
The future vision is a result of the concept of urban sustainability as clarified, advocated, and advanced by many scholars, academics, theorists, and practitioners in the field, and demonstrated in numerous real-world cities across the globe, especially within ecologically advanced nations. According to several rankings, Sweden, Norway, Finland, Germany, and the Netherlands have the highest level of sustainable development practices (e.g, [ 30 ]). However, the development of the novel model for smart sustainable cities of the future is supported by several case studies from Sweden as well as their integration in terms of the planning practices and development strategies through which sustainable urban forms can be achieved. Additionally, this model involves the way instrumentation, datafication, and computerization are opening up dramatically different forms of optimizing and enhancing the performance of such forms, thereby increasing their contribution to the goals of sustainable development. This entails the ways in which the informational landscape of smart cities as underpinned by big data technologies and their novel applications can be integrated with the physical landscape of sustainable cities, and what this implies in regard to increasing their sustainability benefits. The essence of the idea revolves around the need to harness, analyze, and leverage the deluge of urban data that has hitherto been mostly associated with smart cities but has clear synergies in the functioning, planning, and development of sustainable cities in terms of improving, advancing, and maintaining their contribution to sustainability.
The problems and issues that the sustainable city faces today will, especially if its landscape and strategy continues to be extremely fragmented from and weakly connected with those of the smart city at the technical and policy levels, increase in the future with possibly much greater compounding effects due to the rapid urbanization of the world and the mounting challenges of sustainability in a rather increasingly technologized and computerized world. As a scholarly endeavor, the development of the novel model for smart sustainable cities of the future as a holistic approach to city planning and development is primarily aimed at bringing together and interlinking the sustainable city and smart city landscapes and strategies so as to address and overcome a set of challenging problems associated with the existing sustainable urban forms. This requires finding more creative and effective ways of merging sustainability knowledge with advanced technologies to enhance the performance of such forms in the face of urbanization using cutting-edge technologies. This can be accomplished by amalgamating the compact city with the eco-city into one model of sustainable urban form in terms of the underlying typologies and design concepts as planning practices, and then augmenting this model with big data technologies and their novel applications as a set of innovative solutions and sophisticated approaches being offered by the data-driven city. In this respect, city operating system, operations centers, innovation and living labs, and strategic planning and policy offices will handle the activity of generating, processing, and analyzing the data deluge aimed at adopting those innovation solutions and sophisticated approaches in the context of the smart sustainable city. Practical uses and applications in this regard span a range of urban systems and domains in terms of operations, functions, services, designs, strategies, and policies with respect to sustainability.
The future vision has a high expectation on big data technology to deliver the needed solutions and approaches to meet the optimal level of sustainability and enable the built environment to function in a more constructive way than at present in terms of lowering energy consumption, mitigating pollution, and minimizing waste, as well as in terms of improving equity, inclusion, and the quality of life. This is to be determined by whether and the extent to which a given city is currently badging or regenerating itself as, or manifestly planning to be, sustainable or smart sustainable. And what this entails in terms of long-term targets of sustainable development as set by that city, in particular in connection with its design concepts, typologies, spatial organizations, and scale stabilizations as planning practices. In the short term, although big data technology could theoretically help meet the optimal level of sustainability and enable the instrumentation, datafication, and computerization of the built environment towards purposeful urban functioning and planning, this would be difficult and expensive. Nevertheless, the future vision can be feasible because it has to be realized over the long run.
The technological vision is based on the assumption of a full development, integration, and deployment of big data computing and the underpinning technologies which exist today and are likely to become widely available in the years ahead to achieve the sought goals. The incorporation of these advanced technologies into urban environments is supported by their untapped potential for and proven role in overcoming the problems and challenges of urbanization and sustainability. In this respect, big data computing and the underpinning technologies will be determining in the process of redesigning and restructuring urban places to achieve the optimal level of sustainability.
The future vision (see guiding question 2)
The key goal to be necessarily present in any backcasting endeavor is generating the normative alternative for the future and, as related to step 5 which is to be addressed in one of the upcoming papers, analyzing its opportunities, potentials, environmental and social benefits, and other effects.
Taking the prevailing and emerging trends to the extreme with the main expected developments (the outcome of step 2) in mind, we singled out one major societal driver for one scenario: a situation that is most likely to happen in the future:
A scenario where innovations and advancements in big data science and analytics and the underpinning technologies as a disruptive form of science and technology dramatically changes the rules by which society functions on a global scale.
Accordingly, the futures study envisions the smart sustainable city as:
A form for human settlements that will be able to improve, advance, and maintain its contribution to the goals of sustainable development by being pervaded, monitored, understood, and analyzed by advanced ICT. As such, it is to be realised by the planning practices and design strategies pertaining to the most advocated and prevalent models of sustainable urban form as integrated—as well as underpinned by big data computing and the underlying core enabling technologies in terms of the instrumentation, datafication, and analytics of the built environment. Related sophisticated approaches and novel applications will be developed, applied, and enhanced by a number of strategic urban actors, including urban operations centers, urban services agencies, strategic planning and design offices, policymaking bodies, research centers, and innovation and living labs. The main strategic goal of the future model of data–driven smart sustainable urban form is to secure and uphold environmentally sound, socially beneficial, and economically viable development towards achieving sustainability.
In light of the above, envisaging the smart sustainable city of the future focuses on the urban and technological components and how they should be integrated that make the city functions as a smart sustainable entity as well as a social organism. Central to this quest is the idea of big data computing and the underpinning technologies as an advanced form of ICT penetrating wherever and whatever it can of the built environment to improve and sustain the performance of what and how urban stakeholders can envision and enact in terms of new forms of cities with regard to sustainability. Furthermore, advanced ICT comes into play as a response to the commonly held view that cities should be conceived in terms of both urban strategies and processual outcomes of urbanization, which involves questions related to the behavior of inhabitants; the processes of living, consuming, and producing; and the processes of building urban environments—in terms of whether these are sustainable. The underlying assumption is that conceiving cities only in terms of, or accounting only for, urban strategies to make cities more sustainable remains inadequate to achieve the elusive goals of sustainable development.
The three strands of the model for smart Sustainable City of the future (see guiding question 3)
As hinted at above, the novel model for smart sustainable cities of the future, the more detailed version of the future vision, integrates two models of sustainable urban form: the compact city and the eco-city, with the data-driven city. This will result in a holistic approach to urbanism, which is different, to a great extent, from these cities taken separately as existing approaches to urbanism. Worth pointing out, to reiterate, is that the focus of this amalgamation is on the design concepts and typologies characterizing both the compact city (i.e., compactness, density, diversity, mixed-land use, and sustainable transport) and the eco-city (i.e., renewable resources, passive solar design, ecological and cultural diversity, greening, environmental management, and other key environmentally sound policies) together with the innovative solutions and sophisticated approaches being offered by big data technologies and their novel applications for sustainability, which relate to the data-driven city and its components (i.e., urban operating centers, research centers, living labs, and innovation labs). The nature and scope of this amalgamation are to be determined by how and the extent to which the characteristic features of the data-driven city would dovetail with those of the integrated model of sustainable urban form towards producing what can be described as—data-driven smart sustainable urban form. The possible steps to be taken to attain the smart sustainable city of the future as a desired end-point or future vision is rather the object of step 5 of the backcasting approach, which comes after step 4. This step is concerned with the case studies that need to be performed to strengthen the future vision and thus the novel model with empirical investigation. The guiding questions of these two steps are listed in Table 1 .
Furthermore, it must be noted that there are neither real examples of a truly smart sustainable city that have actually been delivered and thus no precedents to reference, nor future-proofing of the big data technology to ensure that it is able to be adapted, modified, and built upon in an effective way over the next 25 years or so in response to the dynamic changes of technology and fast-moving hi-tech industry. Therefore, the planned big data technology solutions must be evaluated through actual implementation and its successfulness in order to outline the actual opportunity pertaining to the improvement and advancement of urban sustainability. Indeed, big data computing and the underpinning technologies intended to support the smart sustainable city of the future are currently evolving along with those experts and professionals who are needed to support and operate them; sustainability objectives, goals, and directives are increasingly being, and should continue to be, supported and facilitated using this advanced technology as much as possible across urban domains in terms of operations, functions, services, designs, strategies, and policies; and citizens and communities must be involved and engaged with big data technology and related platforms on a far broader scale. The road ahead promises to be an exciting one as more cities become aware of the great potential and clear prospect of integrating the smart city and the sustainable city as landscapes and strategies. In the sequel, we describe the three strands that comprise the novel model for smart sustainable cities of the future as hinted at in the description of the vision of the future above.
- Sustainable cities
There are multiple views on what a sustainable city should be or look like and thus various ways of defining or conceptualizing it. Generally, a sustainable city can be understood as a set of approaches into operationalizing sustainable development in, or practically applying the knowledge about sustainability and related technologies to the planning and design of, existing and new cities or districts. It represents an instance of sustainable urban development, a strategic approach to achieving the long-term goals of urban sustainability. Accordingly, it needs to balance between the environmental, social, and economic goals of sustainability as an integrated process. Specifically, as put succinctly by [ 11 ], p. 11), a sustainable city “strives to maximize the efficiency of energy and material use, create a zero-waste system, support renewable energy production and consumption, promote carbon-neutrality and reduce pollution, decrease transport needs and encourage walking and cycling, provide efficient and sustainable transport, preserve ecosystems and green space, emphasize design scalability and spatial proximity, and promote livability and community-oriented human environments.”
There are different instances of sustainable cities as an umbrella term, which are identified as models of sustainable urban forms, including compact cities, eco-cities, sustainable urbanism, green urbanism, new urbanism, and urban containment, with the first two being often advocated as the most sustainable and environmentally sound models [ 13 ]. In addition, Jabareen [ 40 ] ranks compact cities as more sustainable than eco-cities from a conceptual perspective using a thematic analysis. However, the effects of these models are compatible with the goals of sustainable development in terms of transport provision, mobility and accessibility, travel behavior, energy conservation, pollution and waste reduction, economic viability, life quality, and social equity. Furthermore, there are multiple definitions of compact cities and eco-cities in the literature (e.g., [ 40 , 41 , 42 , 43 , 44 , 45 , 54 , 60 , 61 , 64 , 74 ]). These definitions tend to be based on the wider socio-cultural context in which these models of sustainable urban form are embedded in the form of projects and initiatives and related objectives, requirements, resources, and capabilities. In other words, there is a diversity underneath the various uses of the term compact city and eco-city, as well as a convergence or divergence in the way projects and initiatives conceive of what these city approaches should be.
The compact city model
The concept of the compact city became widespread in the early 1990s as a result of the near clinical separation of land uses because of suburban sprawl that had risen the need for mobility, creating an upsurge in automobile use, which in turn caused high levels of air and noise pollution, as well as decaying city centers. In the 1990s, the European Commission highlighted a number of negative trends in urban development in their Green Paper on the Urban Environment [ 24 ], and therefore argued for denser development, mixed land use, and the transformation of former brownfield sites rather than development in open green areas. Fundamentally, the compact city is characterized by high-density and mixed-land use with no sprawl [ 41 , 42 , 80 ] through the intensification of development, i.e., infill, renewal, redevelopment, and so on. It was around the mid-1990s when the research led to the advocacy of combining compactness and mixed-land use [ 40 ]. Mixed-land use should be encouraged in cities [ 21 ]. In addition, the compact city emphasizes spatial diversity, social mix, sustainable transportation (e.g., transit-rich interconnected nodes), as well as high standards of environmental and urban management systems, energy-efficient buildings, closeness to local squares, more space for bikes and pedestrians, and green areas [ 17 , 19 ]. It has been addressed and can be implemented at different levels, namely neighborhood, district, city, metropolitan, and region, and involves many strategies that can avoid all the problems of modernist design in cities by enhancing the underlying environmental, social, and economic justifications and drivers. Neuman [ 54 ] identifies and enumerates the key dimensions of the compact city in Table 2 .
The compact city is more energy efficient and less polluting because people live in close proximity to workplaces, shops, and leisure and service facilities, which enables them to walk, bike, or take transit. This is in turn anticipated to create a better quality of life by creating more social interaction, community spirit, and cultural vitality (Jenks and Jones 2010). Further, travel distances between activities are shortened due to the heterogeneous zoning that enables compatible land uses to locate in close proximity to one another—mixed-land uses. Such zoning primarily reduces the use of automobiles (car dependency) for commuting, leisure, and shopping trips [ 1 , 75 ]. Integrating land use, transport, and environmental planning is key to minimizing the need for travel and to promoting efficient modes of transport [ 67 ]. Transport systems play particularly an important role in the livability of contemporary cities [ 55 ]. The interrelationship between transport, people, and amenities are argued to be the vital elements of the micro-structure of a sustainable city [ 32 ]. Important to note is that population densities are sufficient for supporting local services and businesses [ 80 ] in terms of economic viability. In high-density development, more land is available for green and agricultural areas, public transport services are superior, and the environmental footprint of the non-renewable resource consumption is steady [ 69 ].
In sum, the compact city model has been advocated as more sustainable urban form due to several reasons: “First, compact cities are argued to be efficient for more sustainable modes of transport. Second, compact cities are seen as a sustainable use of land. By reducing sprawl, land in the countryside is preserved and land in towns can be recycled for development. Third, in social terms, compactness and mixed uses are associated with diversity, social cohesion, and cultural development. Some also argue that it is an equitable form because it offers good accessibility. Fourth, compact cities are argued to be economically viable because infrastructure, such as roads and street lighting, can be provided cost-effectively per capita.” ([ 40 ], p. 46).
The eco-city model
The idea of the eco-city is widely varied in conceptualization and operationalization, and also difficult to delineate. According to the most comprehensive survey of eco-cities to date performed by Joss [ 43 ], the diversity and plurality of the projects and initiatives reflected in the use of the term “eco-city” across the globe make it difficult to develop a meaningful definition. Therefore, the concept of the eco-city has taken on many definitions in the literature. Richard Register, an architect widely credited as the first to have coined the term, describes an eco-city as “an urban environmental system in which input (of resources) and output (of waste) are minimized” [ 61 ]. Joss [ 44 ] states that an eco-city must be, using three analytical categories, developed on a substantial scale, occurring across multiple domains, and supported by policy processes. As an umbrella metaphor, the eco-city “encompasses a wide range of urban-ecological proposals that aim to achieve urban sustainability. These approaches propose a wide range of environmental, social, and institutional policies that are directed to managing urban spaces to achieve sustainability. This type promotes the ecological agenda and emphasizes environmental management through a set of institutional and policy tools.” ([ 40 ], p. 47) This implies that realizing an eco-city requires making countless decisions about urban design, governance, sustainable technologies, and so on [ 60 ]. This in turn signifies that the relationship between sustainable development objectives and urban planning interventions is a subject of much debate [ 22 , 79 ].
Irrespective of the way the idea of the eco-city has been conceptualized and operationalized, there are still some criteria that have been proposed to identify what a desirable or ideal “eco-city” is or looks like, comprising the environmental, social and economic goals of sustainable development. Roseland [ 64 ] and Harvey [ 35 ] describe an ideal “eco-city” as a city that fulfills the following requirements:
Operates on a self-contained local economy that obtains resources locally
Maximizes energy and water efficiency, thereby promoting conservation of resources
Manages an ecologically beneficial waste management system that promotes recycling and reuse to create a zero-waste system
Promotes the use and production of renewable energy, thereby being entirely carbon-neutral
Has a well-designed urban city layout that promotes walkability, biking, and the use of public transportation systems
Ensures decent and affordable housing for all socio-economic and ethnic groups and improves jobs opportunities for disadvantaged groups
Supports urban and local farming
Supports future progress and expansion over time.
As added by Graedel [ 33 ], the eco-city is scalable and evolvable in design in response to urban growth and need changes. Based on these characteristic features, the eco-city and green urbanism overlap or share several concepts, ideas, and visions in terms of the role of the city and positive urbanism in shaping more sustainable places, communities, and lifestyles [ 5 ], pp. 6–8, cited in [ 40 ]) views, while arguing for the need for new approaches to urbanism to incorporate more ecologically responsible forms of living and settlement, a city exemplifying green urbanism as one that:
strives to live within its ecological limits;
is designed to function in ways analogous to nature;
strives to achieve a circular rather than a linear metabolism;
strives towards local and regional self-sufficiency;
facilitates more sustainable lifestyles; and
emphasizes a high quality of neighborhood and community life.
The eco-city approaches tend to emphasize different aspects of sustainability, namely passive solar design, greening, sustainable housing, sustainable urban living, and living machines [ 40 ]. Worth noting is that, as a general consensus, the eco-city is eco-amorphous (formless) in terms of typologies, although it emphasizes passive solar and ecological design [ 40 ]. Indeed, it is evident that the form specificities are on less focus in eco-city development. That is to say, the built environment of the city in terms of urban design features and spatial organizations is insignificant, unlike the compact city which focuses on the spatial patterns of physical objects. Rather, what counts most is how the city as a social fabric is organized, managed, and governed. In this line of thinking, [ 70 ], p. 37), state, ‘social, economic, and cultural variables are far more important in determining the good city than any choice of spatial arrangements.’ In view of that, the focus is on the role of different environmental, social, economic, institutional, and land use policies in managing and governing the city to achieve the required level of sustainability (e.g., [ 25 , 40 , 63 ]).
The data-driven city and its smart and sustainable dimensions
“Data-driven smart sustainable cities” is a term that has recently gained traction in academia, government, and industry to describe cities that are increasingly composed and monitored by ICT of ubiquitous and pervasive computing and thus have the ability of using advanced technologies by city operations centers, strategic planning and policy offices, research centers, innovation labs, and living labs for generating, processing, and analyzing the data deluge in order to enhance decision making processes and to develop and implement innovative solutions for improving sustainability, efficiency, resilience, equity, and the quality of life [ 13 ]. It entails developing a citywide instrumented system (i.e., inter-agency control, planning, innovation, and research hubs) for creating and inventing the future. For example, a data-driven city operations center, which is designed to monitor the city as a whole, pulls or brings together real-time data streams from many different agencies spread across various urban domains and then analyze them for decision making and problem solving purposes: optimizing, regulating, and managing urban operations (e.g., traffic, transport, mobility, energy, etc.).
As cities are routinely embedded with all kinds of ICT forms, including infrastructures, platforms, systems, devices, sensors and actuators, and networks, the volume of data generated about them is growing exponentially and diversifying, providing rich, heterogenous streams of information about urban environments and citizens. This data deluge enables a real-time analysis of different urban systems and interconnects data to provide detailed views of the relationships between various forms of data that can be utilized for improving the various aspects of urbanity through new modes of operational functioning, planning, development, and governance in the context of sustainability.
On the evolving integration of data-driven, smart, and sustainable cities
Cities are becoming ever more computationally augmented and digitally instrumented and networked, their systems interlinked and integrated, their domains combined and coordinated, and their networks coupled and interconnected, and consequently, vast troves of urban data are being generated and used to regulate, control, manage, and organize urban life in real time. In other words, the increasing pervasiveness of urban systems, domains, and networks utilizing digital technologies is generating enormous amounts of digital traces capable of reflecting in real time how people make use of urban spaces and infrastructures and how urban activities and processes are performed. This informational asset is being leveraged in steering cities. Indeed, citizens leave their digital traces just about everywhere they go, both voluntarily and involuntarily, and when cross-referenced with each citizen’s spatial, temporal, and geographical contexts, the data harnessed at this scale offers a means of describing, and responding to, the dynamics of the city in real time. In addition to individual citizens, city systems, domains, and networks constitute the main source of data deluge, which is generated by various urban entities, including governmental agencies, authorities, administrators, institutions, organizations, enterprises, and communities by means of urban operations, functions, services, designs, strategies, and policies.
Smart cities are increasingly connecting the ICT infrastructure, the physical infrastructure, the social infrastructure, and the economic infrastructure to leverage their collective intelligence, thereby striving to render themselves more sustainable, efficient, functional, resilient, livable, and equitable. It follows that smart cities of the future seek to solve a fundamental conundrum of cities—ensure sustainable socio-economic development, equity, and enhanced quality-of-life at the same time as reducing costs and increasing resource efficiency and environment and infrastructure resilience. This is increasingly enabled by utilizing a fast-flowing torrent of urban data and the rapidly evolving data analytics technologies; algorithmic planning and governance; and responsive, networked urban systems. In particular, the generation of colossal amounts of data and the development of sophisticated data analytics for understanding, monitoring, probing, regulating, and planning the city are significant aspects of smart cities that are being embraced by sustainable cities to improve, advance, and maintain their contribution to the goals of sustainable development (e.g., [ 8 , 13 , 17 , 18 ]). Indeed, there has recently been much enthusiasm in the domain of smart sustainable urbanism about the immense possibilities and fascinating opportunities created by the data deluge and its extensive sources with regard to optimizing and enhancing urban operational functioning, management, planning, design, and development in line with the goals of sustainable development as a result of thinking about and understanding sustainability and urbanization and their relationships in a data-analytic fashion for the purpose of generating and applying knowledge-driven, fact-based, strategic decisions in relation to such urban domains as transport, traffic, mobility, energy, environment, education, healthcare, public safety, public services, governance, and science and innovation. For supra-national states, national governments, and city officials, smart cities offer the enticing potential of environmental and socio-economic development, and the renewal of urban centers as hubs of innovation and research (e.g., [ 2 , 4 , 13 , 19 , 46 , 51 , 71 ]). While there are several main characteristics of a smart city as evidenced by industry and government literature (e.g., [ 36 , 46 ] for an overview), the one that the futures study, and thus this paper, is concerned with focuses on environmental and social sustainability.
A framework for the data-driven smart sustainable city
The framework for the data-driven smart sustainable city illustrated in Fig. 2 entails specialized urban, technological, organizational, and institutional elements dedicated for improving, advancing, and maintaining the contribution of such city to the goals of sustainable development [ 13 ]. It is derived based on thematic analysis and technical literature. This justifies the relationship between the underlying components. Furthermore, underlying the idea of the data-driven smart sustainable city is the process of drawing all the kinds of analytics associated with urban life into a single hub, supported by the broader public and open data analytics. This involves creating a city-wide instrumented or centralized system that draws together data streams from many agencies (across city domains) for large scale analytics and then direct them to different centers, labs, and offices. Urban operating systems explicitly link together multiple urban technologies to enable greater coordination of urban systems and domains. Urban operations centers attempt to draw together and interlink urban big data to provide integrated and holistic views and synoptic city intelligence through processing, analyzing, visualizing, and monitoring the vast deluge of urban data that can be used for real-time decision-making pertaining to sustainability by means of big data ecosystems. Strategic planning and policy centers serve as a data analytic hub to weave together data from many diverse agencies to control, manage, regulate, and govern urban life more efficiently and effectively in relation to sustainability. This entails an integration that enables systemwide effects to be understood, analyzed, tracked, and built into the very designs and responses that characterize urban operations, functions, and services. As far as research centers and innovation labs are concerned, they are associated with research and innovation for the purpose of developing and disseminating urban intelligence functions. For the anatomy of the data-driven smart sustainable city in terms of digital instrumentation, datafication, computerization, as well as urban operations centers, strategic planning and policy offices, living labs, innovations labs, urban intelligence functions, and so on, the reader can be directed to Bibri [ 15 ].
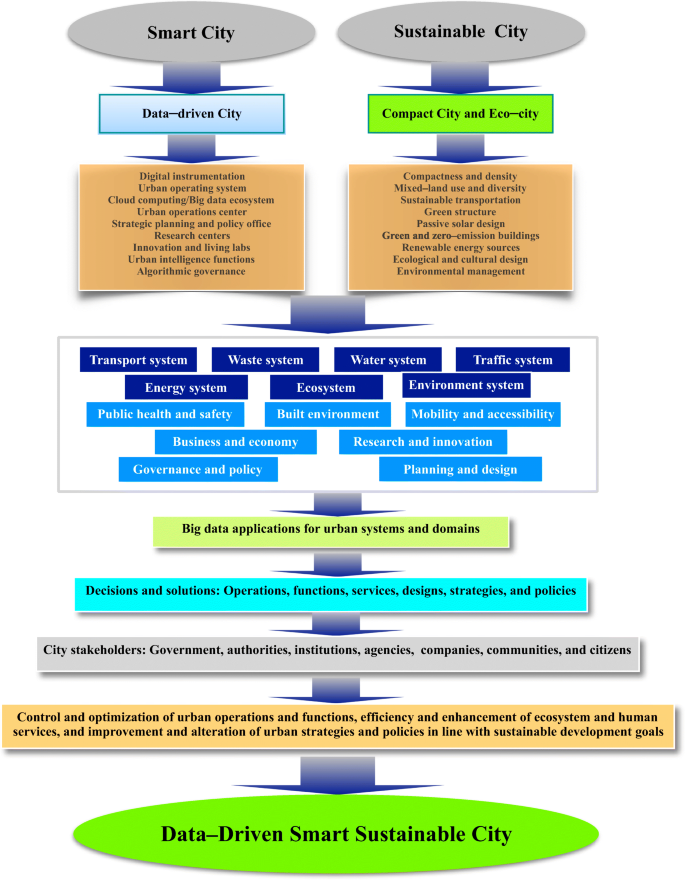
A framework for the data-driven smart sustainable city. Source: Adapted from Bibri [ 15 ]
The rationale behind developing the future vision (see guiding question 4)
The arguments, a set of reasons given in support of the novel model for smart sustainable cities of the future, are compiled and distilled from the outcome of step 2 of the backcasting study conducted by Bibri and Krogstie [ 19 ]. There are many reasons for integrating the existing models of sustainable urban form as a set of practices, or many explanations of controlling the concepts and principles of these practice in the domain of urban sustainability. This applies also to the integration of the sustainable city and the data-driven city as different approaches to urbanism. Here, we identify the key reasons in relevance to the aim of the futures study. This is accordingly to justify the research pursuit of analyzing, investigating, and developing the proposed model for smart sustainable city of the future.
Amalgamating the compact city model with the eco-city model
Being one of the most significant intellectual and practical challenges for three decades, the development of a desirable model of sustainable urban form continues to motivate and inspire collaboration between researchers, academics, and practitioners to create more effective design and planning solutions based on a more integrated and holistic perspective.
Different scholars and planners may develop different combinations of design concepts to achieve the goals of sustainable development. They might come with different forms, where each form emphasizes different concepts and contributes differently to sustainability.
Sustainable urban forms have many overlaps among them in their concepts, ideas, and visions. While there is nothing wrong with such forms being different yet compatible and not mutually exclusive, it can extremely be beneficial and strategic to find innovative ways of combining their distinctive concepts and key differences towards more holistic forms for improving sustainability performance.
Compact cities have a form as they are governed by static planning and design tools, whereas eco-cities are amorphous: without a clearly defined form, thereby the feasibility and potential of their integration into one model that can eventually accelerate sustainable development towards achieving the optimal level of sustainability.
Neither real-world cities nor academics have yet developed convincing models of sustainable urban form, and the components of such form are still not yet fully specified.
More in-depth knowledge on planning practices is needed to capture the vision of sustainable urban development, so too is a deeper understanding of the multi-faceted processes of change to achieve sustainable urban forms. This entails conceptualizing multiple pathways towards attaining this vision and developing a deeper understanding of the interplay between social and technical solutions for sustainable urban forms.
Merging the integrated model of sustainable urban form with the data-driven smart city model
Smart urbanism as being predominately driven by big data computing and the underpinning technologies has recently revived the debate about sustainable cities, and promises to add a whole new dimension to sustainability by enhancing the outcome of the design principles and strategies underlying the existing models of sustainable urban form in ways that enable such form to achieve the optimal level of sustainability.
It is an urban world where the physical landscape of sustainable cities and the informational landscape of smart cities are increasingly being merged. Hence, it is high time for sustainable urban forms to embrace and leverage what data-driven smart cities have to offer in terms of innovative solutions and sophisticated approaches to overcome the complex challenges of sustainability and urbanization.
A large part of research within the emerging area of smart sustainable cities focuses on exploiting the potentials and opportunities of advanced technologies and their novel applications to mitigate or overcome the issue of sustainable cities and smart cities being extremely fragmented as landscapes and weakly connected as approaches, especially at the technical and policy levels.
There is huge potential for using big data computing and the underpinning technologies to advance sustainable urban forms through novel approaches to decision support in the form of intelligence functions enabled by the analytical power of the deluge of urban data.
Tremendous opportunities are available for utilizing big data applications in sustainable cities to optimize and enhance their operations, functions, services, designs, strategies, and policies, as well as to find answers to challenging analytical questions and thereby advance knowledge.
As an integrated and holistic approach, smart sustainable cities tend to take multiple forms of combining the strengths of sustainable cities and smart cities based on how the concept of smart sustainable cities can be conceptualized and operationalized. As a corollary of this, there is a host of unexplored opportunities towards new approaches to smart sustainable urban development.
Problems, issues, and challenges (see guiding question 5)
The issue of sustainable urban forms has always been problematic and daunting to deal with. In view of that, the intellectual challenge to produce a theoretically and practically convincing model of sustainable urban form with clear components continues to induce scholars, academics, planners, scientists, and real-world cities even to create a more successful and robust model of such form. In addition, the contribution of the existing models of sustainable urban form to sustainability has, over the last three decades or so, been subject to much debate, generating a growing level of criticism that essentially questions its practicality, intellectual foundation, and added value.
Developing the model for smart sustainable cities of the future is aimed at improving, advancing, and sustaining the contribution of sustainable urban forms to the goals of sustainable development with support of big data computing and the underpinning technologies as an advanced form of ICT. This is due to the underlying potential for enhancing and optimizing urban operations, functions, designs, services, strategies, and practices in line with the goals of sustainable development, as well as for solving a number of problems, addressing key issues, and overcoming complex challenges in the context of sustainable urban forms. These are distilled and compiled from an extensive interdisciplinary literature review and the outcome of step 2 of the backcasting study ([ 16 , 19 ]) (Table 3 ).
Key novel analytical and practical applications of big data technology for the future vision (see guiding question 6)
Big data applications are increasingly permeating the systems and domains of sustainable cities. This can be seen as a new ethos added to the era of sustainable urbanism in response to the rise of ICT and the spread of urbanization as major global shifts at play today. The characteristic spirit of this era is manifested in the behavior and aspiration of sustainable cities towards embracing what big data computing and the underpinning technologies have to offer in order to bring about sustainable development and thus achieve sustainability under what is labeled “smart sustainable cities of the future.” The range of the emerging big data applications as novel analytical and practical solutions that can be utilized for enhancing the sustainability performance of sustainable cities is potentially huge. A recent study conducted by Bibri [ 13 ] reveals that tremendous opportunities are available for utilizing big data applications to improve, advance, and maintain the contribution of sustainable cities to the goals of sustainable development. This finding is based on identifying, synthesizing, distilling, and enumerating the most common big data applications in relation to a number of urban domains or sub-domains, as well as elucidating their sustainability effects associated with the underlying functionalities pertaining to these domains or sub-domains. These specifically include transport and traffic, mobility, energy, power grid, environment, buildings, infrastructures, urban planning, urban design, governance, healthcare, education, public safety, and academic and scientific research.
The potential of big data technology lies in enabling sustainable cities to harness and leverage their informational landscape in effectively understanding, monitoring, probing, and planning their systems in ways that enable them to achieve the optimal level of sustainability. To put it differently, the use of this advanced technology is projected to play a significant role in realizing the key characteristic features of such cities, namely the efficiency of operations and functions, the efficient utilization of natural resources, the intelligent management of infrastructures and facilities, the lowering of pollution and waste, the improvement of the quality of life and well-being of citizens, and the enhancement of mobility and accessibility.
Discussion and conclusion
Long-lasting and substantive transformations such as sustainability transitions can only come about through the accumulation of several integrated smaller-scale actions associated with strategically successful initiatives and programs. The backcasting approach to futures studies can help to highlight such initiatives and programs, and also play a key role in sustaining the momentum in the quest to bring about major transformations. In the context of city planning and development, this approach can be used to illustrate what might happen to cities in order to allow them to adapt to perceived future trends and to manage uncertainty. As such, it aids in dealing with this uncertainty by clarifying what the most desirable possibilities are, what can be known, what is already known, as well as how today’s decisions may play out in each of a variety of plausible futures. Futures studies using backcasting approaches allow for a better understanding of future opportunities and exploring the implications of alternative development paths that can be relied on to avoid the impacts of the future. There is a strong belief that future-orientated planning can change development paths. The interest in the future of smart sustainable cities is driven by the aspiration to transform the continued urban development path. Therefore, it is worthy to venture some thoughts about where it might be useful to channel the efforts now and in the future in relation to smart sustainable urban planning and development. The backcasting scenario, a description of possible actions in the future, starts with constructing the vision of the future and then works backwards in time step-by-step to figure out how this future could emerge as a particular “desired end-point” through identifying the necessary steps to reach it.
This paper aimed to generate a vision for smart sustainable cities of the future by answering the 6 guiding questions for step 3 of the futures study being conducted. We described the terms of reference for the future vision under the visionary approach. These terms entail the scope and limitation of the area of knowledge to be focused on and the description of the structure and objectives of the futures study. Then, we described how the future vision look like, more specifically, the novel model for smart sustainable cities of the future and its role in achieving the optimal level of sustainability. Following this, we detailed how the proposed model is different from existing approaches to urbanism, namely compact cities, eco-cities, data-driven smart cities by describing and discussing the three strands that comprise this model, as well as how they intertwine with one another in the context of sustainability. This was justified by providing the rationale for developing the future vision, which represents the short and concise version of the respective model. Of particular importance, we provided a tabulation version of the review and discussion of the sustainability problems and issues that are supposed to be tackled by meeting the objectives stated and thus achieving the goals specified in step 1 of the backcasting study. In relation to this, we provided an account of the kind of technologies and their novel applications that are intended to be used as part of the proposed model.
Working with a long-term image of the future is meant to increase the possibilities of, and accelerate the movement towards, reaching a smart sustainable city. In this regard, the novel model for smart sustainable cities of the future will be the boost to new forms of policy analysis and planning in the era of big data revolution, and the greatest impacts of big data technology will be on the way we improve, advance, and maintain the contribution of sustainable cities to the goals of sustainable development in the future by means of integrating urban strategies and technological innovations. The main goal of big data technology is to provide intelligence functions that will make this possible in the most effective ways.
Worth pointing out is that smart sustainable cities as an integrated model take multiple forms of combining the strengths of sustainable cities and smart cities based on how the concept of smart sustainable cities can be conceptualized and operationalized. Just as it has been the case for sustainable cities: there are multiple visions of, and pathways towards achieving, sustainable urban development. As a corollary of this, there is a host of unexplored opportunities towards new approaches to smart sustainable urban planning and development. These future endeavors reflect the characteristic spirit and prevailing tendency of the ICT-sustainability-urbanization era as manifested in its aspirations for directing the advances in ICT of pervasive computing towards addressing and overcoming the challenges of sustainability and urbanization in the defining context of smart sustainable cities of the future.
Similarly, in relation to backcasting as a planning approach, multiple visions can be used to explore different future alternatives as to smart sustainable cities. It is important, though, to take into consideration that big data technologies as part of future visions seem to be de-urbanized in the sense of not being made to work within a particular urban context, or to be tailored to different urban landscapes and strategies. Besides, it is unfeasible simply to plop down advanced technologies and force them to work in a given urban space. Cities are so characterized by key specificities such that technology systems might work in one city and not be desirable in another, unless they are dramatically reworked or reshaped to be practical in those cities where they have to be implemented. Hence, there is a need for urbanizing big data technologies and in different directions, we content and advocate, when it comes to generating future visions. With that in mind, the future vision this paper is concerned with pertains to cities in ecologically and technologically advanced nations.
Availability of data and materials
Not applicable
Alberti M (2000) Urban form and ecosystem dynamics: empirical evidence and practical implications. In: Williams K, Burton E, Jenks M (eds) Achieving sustainable urban form. E & FN Spon, London, pp 84–96
Google Scholar
Al Nuaimi E, Al Neyadi H, Nader M, Al-Jaroodi J (2015) Applications of big data to smart cities. J Internet Serv Appl 6(25):1–15
Angelidou M, Psaltoglou A, Komninos N, Kakderi C, Tsarchopoulos P, Panori A (2017) Enhancing sustainable urban development through smart city applications. J Sci Technol Policy Manage:1–25
Batty M, Axhausen KW, Giannotti F, Pozdnoukhov A, Bazzani A, Wachowicz M, Ouzounis G, Portugali Y (2012) Smart cities of the future. Eur Phys J 214:481–518
Beatley T (2000) Green urbanism: learning from European cities. Island Press, Washington, DC
Bettencourt LMA (2014) The uses of big data in cities. Santa Fe Institute, Santa Fe
Book Google Scholar
Bibri SE (2018a) Smart sustainable cities of the future: the untapped potential of big data analytics and context aware computing for advancing sustainability. Springer, Berlin
Bibri SE (2018b) The IoT for smart sustainable cities of the future: an analytical framework for sensor–based big data applications for environmental sustainability. Sustain Cities and Soc 38:230–253
Article Google Scholar
Bibri SE (2018c) A foundational framework for smart sustainable city development: theoretical, disciplinary, and discursive dimensions and their synergies. Sustain Cities Soc 38:758–794
Bibri SE (2018d) Backcasting in futures studies: a synthesized scholarly and planning approach to strategic smart sustainable city development. Eur J Future Res:2–27
Bibri SE, Krogstie J (2017) The core enabling technologies of big data analytics and context-aware computing for smart sustainable cities: a review and synthesis. J Big Big Data 4(38):1–50
Bibri SE (2019a) On the sustainability of smart and smarter cities in the era of big data: an interdisciplinary and transdisciplinary literature review. J Big Data 6(25):2–64
Bibri SE (2019b) Big data science and analytics for smart sustainable urbanism: unprecedented paradigmatic shifts and practical advancements. Springer, Germany, Berlin
Bibri SE (2019c) The Sciences Underlying Smart Sustainable Urbanism: Unprecedented Paradigmatic and Scholarly Shifts in Light of Big Data Science and Analytics. Smart Cities 2(2):179–213
Bibri SE (2019d) The anatomy of the data-driven smart sustainable city: instrumentation, datafication, computerization and related applications. J Big Data 6:59
Bibri SE, Krogstie J (2017a) Smart sustainable cities of the future: an extensive interdisciplinary literature review. Sustain Cities Soc 31:183–212
Bibri SE, Krogstie J (2017b) ICT of the new wave of computing for sustainable urban forms: their big data and context-aware augmented typologies and design concepts. Sustain Cities Soc 32:449–474
Bibri SE, Krogstie J (2018) The big data deluge for transforming the knowledge of smart sustainable cities: a data mining framework for urban analytics, proceedings of the 3d annual international conference on smart city applications. ACM, Tetouan
Bibri SE, Krogstie J (2019) A Scholarly Backcasting Approach to a Novel Model for Smart Sustainable Cities of the Future: Strategic Problem Orientation, Journal of City, Territory, and Architecture (in press).
Bifulco F, Tregua M, Amitrano CC, D’Auria A (2016) ICT and sustainability in smart cities management. Int J Public Sect Manage 29(2):132–147
Breheny M (ed) (1992) Sustainable development and urban form. Pion, London
Bulkeley H, Betsill M (2005) Rethinking sustainable cities: multilevel governance and the “urban” politics of climate change. Environ Politics 14(1):42–63
Carlsson-Kanyama A, Dreborg KH, Eenkhorn BR, Engström R, Falkena B (2003) Image of everyday life in the future sustainable city: experiences of back-casting with stakeholders in five European cities. The Environmental Strategies Research Group (Fms)—report 182, The Royal Institute of Technology, Stockholm, Sweden, 2003. Report available at/react-text
CEC (1990) Green paper on the urban environment – communication from the Commission to the Council and the Parliament. Commission of the European Communities (CEC), Brussels
Council of Europe (1993) The European urban charter—standing conference of local and regional authorities of Europe.
David D (2017) Environment and urbanization. Int Encyclopedia Geogr 24(1):31–46. https://doi.org/10.1002/9781118786352.wbieg0623
Dempsey N, Jenks M (2010) The future of the compact city. Built Environ 36(1):116–121
De Roo G (2000) Environmental conflicts in compact cities: complexity, decision - making, and policy approaches. Environment and Planning B: Planning and Design 27:151–162
Dreborg KH (1996) Essence of backcasting. Futures 28(9):813–828
Dryzek JS (2005) The politics of the earth: environmental discourses 2nd ed. Oxford University Press, Oxford
Estevez E, Lopes NV, Janowski T (2016) Smart sustainable cities. Reconnaissance Study 330
Frey H (1999) Designing the city: towards a more sustainable urban form. E & FN Spon, London
Graedel T (2011) Industrial ecology and the ecocity. National Academy of engineering
Han J, Meng X, Zhou X, Yi B, Liu M, Xiang W-N (2016) A long–term analysis of urbanization process, landscape change, and carbon sources and sinks: a case study in China’s Yangtze River Delta region. J Clean Prod 141:1040–1050. https://doi.org/10.1016/j.jclepro.2016.09.177
Harvey F (2011) Green vision: the search for the ideal eco-city. Financ Times, London
Hollands RG (2008) Will the real smart city please stand up? City Anal Urban Trends Cult Theory Policy Action 12(3):303–320
Holmberg J (1998) Backcasting: a natural step in operationalizing sustainable development. Greener Manage Int (GMI) 23:30–51
Holmberg J, Robèrt KH (2000) Backcasting from non-overlapping sustainability principles: a framework for strategic planning. Int J Sustain Dev World Ecol 7(4):291–308
Höjer M (2000) What is the point of IT? Backcasting urban transport and land-use futures. Doctoral dissertation, Department of Infrastructure and Planning. The Royal Institute of Technology, Stockholm
Jabareen YR (2006) Sustainable urban forms: their typologies, models, and concepts. J Plann Educ Res 26:38–52
Jenks M, Burton E, Williams K (1996a) A sustainable future through the compact city? Urban intensification in the United Kingdom. Environ Des 1(1):5–20
Jenks M, Burton E, Williams K (eds) (1996b) The compact city: a sustainable urban form? E&FN Spon Press, London
Joss S (2010) Eco-cities—a global survey 2009. WIT Trans Ecol Environ 129:239–250
Joss S (2011) Eco-cities: the mainstreaming of urban sustainability; key characteristics and driving factors. Int J Sustain Dev Plan 6(3):268–285
Joss S, Cowley R, Tomozeiu D (2013) Towards the ubiquitous eco-city: an analysis of the internationalisation of eco-city policy and practice. J Urban Res Pract 76:16–22
Kitchin R (2014) The real–time city? Big data and smart urbanism. Geo J 79:1–14
Kitchin R (2015) Data–Driven. Networked Urbanism. https://doi.org/10.2139/ssrn.2641802
Kitchin R (2016) The ethics of smart cities and urban science. Phil Trans R Soc A 374:20160115
Kramers A, Höjer M, Lövehagen N, Wangel J (2014) Smart sustainable cities: exploring ICT solutions for reduced energy use in cities. Environ Model Softw 56:52–62
Kramers A, Wangel J, Höjer M (2016) Governing the smart sustainable city: the case of the Stockholm Royal Seaport. In: Proceedings of ICT for sustainability 2016, vol 46. Atlantis Press, Amsterdam, pp 99–108
Kourtit K, Nijkamp P, Arribas-Bel D (2012) Smart cities perspective—a comparative European study by means of self-organizing maps. Innovation 25(2):229–246
Miola A (2008) Backcasting approach for sustainable mobility. European Commission, Joint Research Centre, Institute for Environment and Sustainability
Neirotti P, De Marco A, Cagliano AC, Mangano G, Scorrano F (2014) Current trends in smart city initiatives—some stylized facts. Cities 38:25–36
Neuman M (2005) The compact city fallacy. J Plan Educ Res 25:11–26
Newman P (2000) Urban form and environmental performance. In: Williams K, Burton E, Jenks M (eds) Achieving sustainable urban. E & FN Spon, London, pp 46–53
Quist J, Rammelt C, Overschie M, de Werk G (2006) Backcasting for sustainability in engineering education: the case of Delft University of Technology. J Clean Prod 14:868–876
Quist J, Knot M, Young W, Green K, Vergragt P (2001) Strategies towards sustainable households using stakeholder workshops and scenarios. Int J Sustain Dev 4:75–89
Pantelis K, Aija L (2013) Understanding the value of (big) data. In: Big data 2013 IEEE international conference on IEEE, pp 38–42
Chapter Google Scholar
Phdungsilp A (2011) Futures studies’ backcasting method used for strategic sustainable city planning. Futures 43(7):707–714
Rapoport E, Vernay AL (2011) Defining the eco-city: a discursive approach. In: Paper presented at the management and innovation for a sustainable built environment conference, international eco-cities initiative. The Netherlands, Amsterdam, pp 1–15
Register R (2002) Eco-cities: building cities in balance with nature. Berkeley Hills Books, Berkeley, CA
Rivera MB, Eriksson E, Wangel J (2015) ICT practices in smart sustainable cities—in the intersection of technological solutions and practices of everyday life. In: 29th international conference on informatics for environmental protection (EnviroInfo 2015), third international conference on ICT for sustainability (ICT4S 2015). Atlantis press, The Netherlands, pp 317–324
Robinson J, Tinker J (1998) Reconciling ecological, economic and social imperatives. In: Schnurr J, Holtz S (eds) The cornerstone of development: integrating environmental, social, and economic policies. IDRC International Development Research Center and Lewis Publishers, Ottawa, pp 9–43
Roseland M (1997) Dimensions of the eco-city. Cities 14(4):197–202
Rotmans J et al (2000) Visions for a sustainable Europe. Futures 32(2000):809–831
Roth A, Kaberger T (2002) Making transport sustainable. J Clean Prod 10:361–371
Sev A (2009) How can the construction industry contribute to sustainable development? A conceptual framework. Sustain Dev 17:161–173
Shahrokni H, Årman L, Lazarevic D, Nilsson A, Brandt N (2015) Implementing smart urban metabolism in the Stockholm Royal Seaport: smart city SRS. J Ind Ecol 19(5):917–929
Suzuki, H, Dastur, A, Moffatt, S, Yabuki, N, Maruyama, H. (2010) Eco2 cities – ecological cities as economic cities. The World Bank
Talen E, Ellis C (2002) Beyond relativism: reclaiming the search for good city form. J Plann Edu Res 22:36–49
Townsend A (2013) Smart cities—big data, civic hackers and the quest for a new utopia. Norton & Company, New York
Tuominent P, Tapio T, Jarvi, Banister D (2014) Pluralistic backcasting: Integrating multiple visions with policy packages for transport climate policy. Futures 60:41–58
United Nations (2015) Big Data and the 2030 agenda for sustainable development. Prepared by A. Maaroof.
Van Bueren E, van Bohemen H, Itard L, Visscher H (2011) Sustainable urban environments: an ecosystem approach. International Publishing, Springer
Van U-P, Senior M (2000) The contribution of mixed land uses to sustainable travel in cities. In: Williams K, Burton E, Jenks M (eds) Achieving sustainable urban form. E & FN Spon, London, pp 139–148
Weaver P, Jansen L, van Grootveld G, van Spiegel E, Vergragt P (2000) Sustainable technology development. Greenleaf Publishers, Sheffield
Wheeler SM, Beatley T (eds) (2010) The sustainable urban development reader. Routledge, London, New York
Whitehead M (2003) (Re)Analysing the Sustainable City: Nature, Urbanism and the Regulation of Socio-Environmental Relations in the UK. Urban Studies 40(7):1183–1206
Williams K (2009) Sustainable cities: research and practice challenges. Int J Urban Sustain Dev 1(1):128–132
Williams K, Burton E, Jenks M (eds) (2000) Achieving sustainable urban form. E & FN Spon, London
Yigitcanlar T, Lee SH (2013) Korean ubiquitous-eco-city: a smart-sustainable urban form or a branding hoax? J. Tech For Soc Ch 89:100–114
Download references
Acknowledgements
Not Applicable.
Author information
Authors and affiliations.
Department of Computer Science, Norwegian University of Science and Technology (NTNU), Sem Saelands veie 9, NO-7491, Trondheim, Norway
Simon Elias Bibri & John Krogstie
Department of Architecture and Planning, Alfred Getz vei 3, Sentralbygg 1, 5th floor, NO-7491, Trondheim, Norway
Simon Elias Bibri
You can also search for this author in PubMed Google Scholar
Contributions
Both authors read and approved the final manuscript.
Author’s information
Simon Elias Bibri is a Ph.D scholar in the area of data-driven smart sustainable cities of the future and Assistant Professor at the Norwegian University of Science and Technology (NTNU), Department of Computer Science and Department of Architecture and Planning, Trondheim, Norway. He holds the following degrees:
Bachelor of Science in computer engineering with a major in software development and computer networks
Master of Science—research focused—in computer science with a major in Ambient Intelligence
Master of Science in computer science with a major in informatics
Master of Science in computer and systems sciences with a major in decision support and risk analysis
Master of Science in entrepreneurship and innovation with a major in new venture creation
Master of Science in strategic leadership towards sustainability
Master of Science in sustainable urban development
Master of Science in environmental science with a major in ecotechnology and sustainable development
Master of Social Science with a major in business administration (MBA)
Master of Arts in communication and media for social change
Postgraduate degree (1 year of Master courses) in management and economics
PhD in computer science and urban planning with a major in data-driven smart sustainable cities
Bibri has earned all his Master’s degrees from different Swedish universities, namely Lund University, West University, Blekinge Institute of Technology, Malmö University, Stockholm University, and Mid–Sweden University.
His current research interests include smart sustainable cities, sustainable cities, smart cities, urban science, urban analytics, sustainability science, complexity science, data-intensive science, data-driven and scientific urbanism, as well as big data computing and its core enabling and driving technologies, namely sensor technologies, data processing platforms, big data applications, cloud and fog computing infrastructures, and wireless communication networks.
Bibri has authored four academic books whose titles are as follows:
The Human Face of Ambient Intelligence: Cognitive, Emotional, Affective, Behavioral and Conversational Aspects (525 pages), Springer, 07/2015.
The Shaping of Ambient Intelligence and the Internet of Things: Historico-epistemic, Socio-cultural, Politico-institutional and Eco-environmental Dimensions (301 pages), Springer, 11/2015.
Smart Sustainable Cities of the Future: The Untapped Potential of Big Data Analytics and Context-Aware Computing for Advancing Sustainability (660 pages), Springer, 03/2018.
Big Data Science and Analytics for Smart Sustainable Urbanism: Unprecedented Paradigmatic Shifts and Practical Advancements (505 pages), Springer, 06/2019.
Corresponding author
Correspondence to Simon Elias Bibri .
Ethics declarations
Ethics approval and consent to participate.
Not Applicable
Consent for publication
Competing interests.
The authors declare that they have no competing interests.
Additional information
Publisher’s note.
Springer Nature remains neutral with regard to jurisdictional claims in published maps and institutional affiliations.
Rights and permissions
Open Access This article is distributed under the terms of the Creative Commons Attribution 4.0 International License ( http://creativecommons.org/licenses/by/4.0/ ), which permits unrestricted use, distribution, and reproduction in any medium, provided you give appropriate credit to the original author(s) and the source, provide a link to the Creative Commons license, and indicate if changes were made.
Reprints and permissions
About this article
Cite this article.
Bibri, S.E., Krogstie, J. Generating a vision for smart sustainable cities of the future: a scholarly backcasting approach. Eur J Futures Res 7 , 5 (2019). https://doi.org/10.1186/s40309-019-0157-0
Download citation
Received : 14 February 2019
Accepted : 18 July 2019
Published : 28 August 2019
DOI : https://doi.org/10.1186/s40309-019-0157-0
Share this article
Anyone you share the following link with will be able to read this content:
Sorry, a shareable link is not currently available for this article.
Provided by the Springer Nature SharedIt content-sharing initiative
- Smart sustainable cities
- Smart cities
- Compact cities
- Data-driven cities
- Big data computing and the underpinning technologies
- Future vision
- Backcasting
- Futures studies
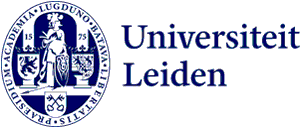
Thesis and Thesis Seminar: Sustainable City B
Admission requirements.
This course is only available for students in the BA Urban Studies, who have obtained 100 EC of the Urban Studies programme, including two Methodological and two Thematic Electives. To follow this thesis seminar, you must have completed the Sustainable City Thematic Elective and you must have completed the Methodological Elective Inferential Statistics.
Description
Thesis seminar.
The Thesis seminar will be centered around a broader topic within the theme (The Multicultural City, The Safe City, The Healthy City and The Sustainable City). This course description will be updates as soon as this topic is known. The focus of the seminars is informed by the themes of the Urban Studies programme: The Multicultural City, The Safe City, The Healthy City and The Sustainable City. After an introduction on a theme relevant to urban studies, students will reflect on their own topic of research, choose their own approach, and consider their topic of research from a multidisciplinary perspective (see below under general academic skills).
The Thesis Seminar provides collective and individual supervision and feedback, by teaching staff and peers. The aim is to guide students through the process of designing a research question or hypothesis and appropriate research method(s), collecting and analyzing literature and writing a literature review, and collecting other research data and materials that are necessary for answering research question or hypothesis .
The exact set-up of the seminars may vary, due to the nature of the research theme, the teaching approach of the seminar leader, and the number and interests of the students. The Thesis Seminar culminates in a Thesis Proposal, which contains a problem definition and research question or hypothesis, theoretical and methodological considerations, a literature overview and a time frame for the thesis research.
The Sustainable city
Sustainable City Thesis Seminar: Towards circular and climate neutral cities
The metabolism of the city (Urban Metabolism) largely determines the sustainability of cities and city-dwellers. For future cities we need to move towards a circular city in which materials are reused and recycled. Cities will also need to be much more self-sufficient if it comes to the supply of energy and water. Rooftop solar panels, heat pumps and zero-energy buildings, smart grids and car-as-powerplant concepts will all contribute to this. Meanwhile, cities house more than half of the people in the world and account for more than two-thirds of global CO2 emissions. Rapid transformation across business models and policy is needed to achieve an integrated net zero carbon future. The IE seminar will focus on topics that contribute to a circular and climate neutral transition in cities. This seminar will provide examples of quantitative tools (e.g., life cycle assessment, and material flow analysis) to determine the socio-economic and environmental impacts of a circular and energy transition in cities. Potential USTS-IE topics are:
Quantification of the household waste generation and the potential of smart logistics on value recovery, resource-efficiency, and climate mitigation
Key factors for the realization of green roof systems
Socio-economic and environmental impacts of repair cafés
Opportunities and barriers to accelerate urban transitions to a net zero future
The bachelor’s Thesis is the final and most important written assignment of the programme. It builds on exercises in essay-writing throughout the curriculum. The bachelor’s Thesis is a research paper of 10,000 words (margins between 9,000 and 11,000 words), excluding front page, table of contents, footnotes and bibliography, which is the result of independent research and writing.
Apart from collective supervision during the seminar, students will receive individual guidance, specifically focused on the subject of their research. The Thesis Seminar lecturer provides this guidance. Students will have four individual meetings with their supervisor during the semester.
Deadline for the submission of the Thesis is June 9 2023 .
Course objectives
General learning outcomes.
See tab Additional information for the overview of the programme's general learning outcomes. In the assessment methods below is outlined which general learning outcome will be tested through which method.
Course objectives, pertaining to the Thesis Seminar and Thesis
1) work with research techniques that are current in the discipline(s) applied 2) analyze and comprehend relevant academic debates 3) report on their studies and research in properly written English 4) participate in debates in an active, prepared and informed way, respecting other people’s convictions and ideas.
The timetables are available through My Timetable .
Mode of instruction
- Tutorial (compulsory attendance) This means that students have to attend every session of the course. If a student is unable to attend a tutorial, they should inform the lecturer in advance, providing a valid reason for absence. The teacher will determine if and how the missed session can be compensated by an additional assignment. If they are absent from a tutorial without a valid reason, they can be excluded from the final exam in the course.
Assessment method
Written Thesis (9.000 – 11.000 words, excluding front page, table of contents, bibliography and foot- or endnotes) -measured general learning outcomes: 1-2, 4-11, 13-21, 25-26 -measured course objectives: 1-4
Thesis proposal (750 – 800 words, excluding bibliography and foot- or endnotes) -measured general learning outcomes: 1-2, 4-11, 13-21, 25-26 -measured course objectives: 1-4
Participation during Thesis Seminars -measured general learning outcomes: 1-2, 4-5, 8-9, 13-26 -measured course objectives: 4
To successfully complete the course, please take note of the following:
The grade for the Thesis Seminar is determined by the Thesis grade.
The grade for the Thesis needs to be a 6.0 or higher.
Students who have been active participants in class and submitted the Thesis on time but scored an overall insuffient mark are entitled to a resit. For the resit, the students are given a chance to hand in an improved version of the Thesis based on the feedback from the Thesis Assessment Form. The deadline for resubmission is to be consulted with the thesis supervisor. In case the improved Thesis is still deemed insufficient, the student must contact the Coordinator of Studies to discuss further possibilities.
Reading list
To be announced.
Registration
- Enrolment through My Studymap is mandatory.
For substantive questions, contact the lecturer listed in the right information bar.
For questions about enrolment, admission, etc, contact the Education Administration Office: Student Affairs Office for BA Urban Studies .
Registration through MyStudyMap is mandatory. Registering in MyStudyMap means placement on the waiting list of that specific Thesis Seminar. The Administration Office will check whether you meet the entry requirements. In case you do not meet the requirements you can be moved to another Thesis Seminar. The Administration Office will place students on a first come first served base. You can only register for one Thesis Seminar.
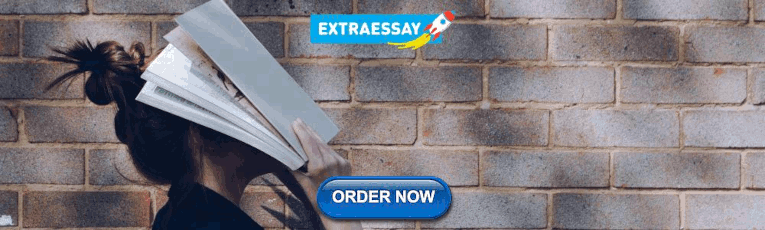
IMAGES
VIDEO
COMMENTS
Sustainable cities have been the leading global paradigm of urbanism (e.g., [1, 31, 149, 221, 222]) thanks to the models of sustainable urban form proposed as new frameworks for redesigning and restructuring urban places for making urban living more sustainable. Compact cities and eco-cities are the most advocated models of sustainable urban ...
Abstract: The article delivers the results of systematic literature review of research on smart. 8. and sustainable cities. An overview of definitions, dimensions and components of smart cit y, 9 ...
governance. The paper has three key goals: (1) to examine how climate change is. affecting urban areas; (2) to assess how cities can enhance urban sustainability by. addressing climate change; (3) to discuss resources available for city leaders wanting to. transition to a sustainable city.
SUST AINABLE CITIES AND C OMMUNITIES. Cities consist of large complex systems that humanity has developed to modify its. environment to thrive; however, the climate crisis and the COVID-19 ...
Therefore, both urban development and enhancing a sustainable quality of life for citizens are at the core of the process of building smart and sustainable cities (Glasmeier & Christopherspn, 2015). As urban revolution is being witnessed, there is an ever-growing demand for smart governance, smart infrastructure, smart mobility, smart economy ...
The fast growth of the urban population increases the demand for energy, water, and transportation, amongst other needs. This study explores the current state and future scenarios of Smart Cities and the environmental, economic, and social challenges that must be overcome. We used the Rapid Review method to understand the challenges of implementing Smart Cities in different urban contexts and ...
Thus, ISO 37122:2019 "Sustainable Cities and Communities—Indicators for smart cities" establishes a series of integrated and interconnected strategies and systems to provide better services and increase quality of life [38,39,40], ensuring equal opportunities for all, protecting the environment, and including some development factors [41 ...
This chapter outlines the vision for a sustainable, resilient, and livable city in the wake of mega risks—which have unleashed forces that have the potential to lead to relatively long-lasting transformation of cities at least in the short term. As the world enters the third year of the pandemic, it is very clear that the COVID-19 crisis is ...
Smart Cities have emerged as one response to the challenges and opportunities created by rapid urbanization. This report presents the results of a reconnaissance study examining the thesis that Smart Cities advance sustainable development. The study analysed 876 scientific publications, recommendations
However, the foundations of the Smart city concept were laid only in the 90s of the 20th century, when sustainability began to be enforced in urban planning, an example can be the Kyoto Protocol ...
Doctoral Thesis in Planning and Decision Analysis With specialization in Urban and Regional Studies KTH Royal Institute of Technology Stockholm, Sweden, 2017 ... 'Sustainable cities and communities' is one of these goals; to make cities inclusive, safe, resilient and sustainable. At the UN conference on housing and sustainable urban ...
Thesis: Ph. D. in Urban and Regional Planning, Massachusetts Institute of Technology, Department of Urban Studies and Planning, 2018. ... Research on sustainable cities has typically emphasized how this change ought to be achieved, outlining specific interventions in the form of new policies and technologies. Problematically, we know far less ...
Safe, Attractive, and Sustainable Cities: A mobility-oriented approach Master's Thesis Author: James Speirs SCIPER N°: 276118 ... While these are essential to any thesis, they do not make up the bulk of resources for this particular paper. This is not the result of their being lacking information. On the contrary, this is because
Di Fan. The last two decades have witnessed a surge in interest in the smart-sustainable city, but it remains unclear how smart cities can achieve the multifaceted goals of sustainable development, such as ecological performance, human development and sustainability efficiency. This study performs a fuzzy-set qualitative comparative analysis ...
with "smart cities" or "sustainable cities." According to the declaration of the World Ecocity Summit 2008 in San Francisco, an ecocity: …is an ecologically healthy city. Into the deep future, the cities in which we live must enable people to thrive in harmony with nature and achieve sustainable development.
The development of the Internet of Things (IoT) technology and their integration in smart cities have changed the way we work and live, and enriched our society. However, IoT technologies present several challenges such as increases in energy consumption, and produces toxic pollution as well as E-waste in smart cities. Smart city applications must be environmentally-friendly, hence require a ...
It is strongly believed that developing more sustainable cities is not just about improving the abiotic and biotic aspects of urban life, it is also about the social aspects of city life, that is—among others—about people's satisfaction, experiences and perceptions of the quality of their everyday environments (see also Beer, 1994).In the context of this study, the relation between urban ...
well-being of residents and the city seems to depend on sustainable and smart decisions. In this study, 18 sustainable urban unit strategies and guidelines, including 8 sustainable. cities, 8 ...
Sustainable cities have been the leading global paradigm of urbanism. Undoubtedly, sustainable development has, since its widespread diffusion in the early 1990s, positively influenced city planning and development. This pertains to the immense opportunities that have been explored and the enormous benefits that have been realized in relation to sustainable urban forms, especially compact ...
The report "Smart Sustainable Cities - Reconnaissance Study" presents the results of a study, conducted by UNU-EGOV and funded by IDRC, that examined the thesis that Smart Cities advance sustainable development. The study analysed 876 scientific publications, recommendations from 51 think tank organizations and 119 concrete Smart City ...
An Interdisciplinary Approach to the Blue Economy Amid the Climate Crisis: Digitization, Urbanization, and Infrastructure Transformation. Aligned with UN Sustainable Development Goals, this journal explores innovations in our approach to future urban living, to address the challenges cities are facing.
Abstract: In order to create a sustainable future for the urban environment in s=Smart cities, it is. necessary to develop a concept of urban transport, partially reduce the use of traditional ...
The Sustainable city. Sustainable City Thesis Seminar: Towards circular and climate neutral cities. The metabolism of the city (Urban Metabolism) largely determines the sustainability of cities and city-dwellers. For future cities we need to move towards a circular city in which materials are reused and recycled. Cities will also need to be ...