Enter your email to subscribe to our newsletter! We have some big things coming and you don't want to miss out. 🤯🚀
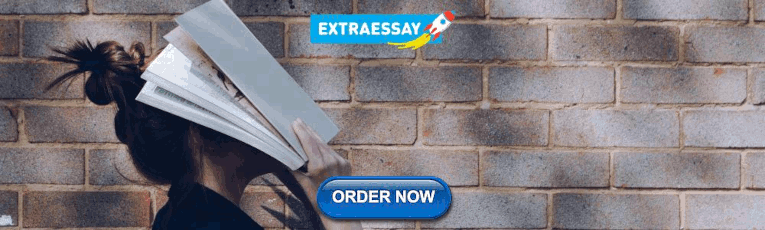
Scale of the Universe
Scale of Universe is an interactive experience to inspire people to learn about the vast ranges of the visible and invisible world.
Click on objects to learn more.
Use the scroll bar to zoom in and out.
Originally created by Cary Huang
Remastered by Dave Caruso , Ben Plate , and more.
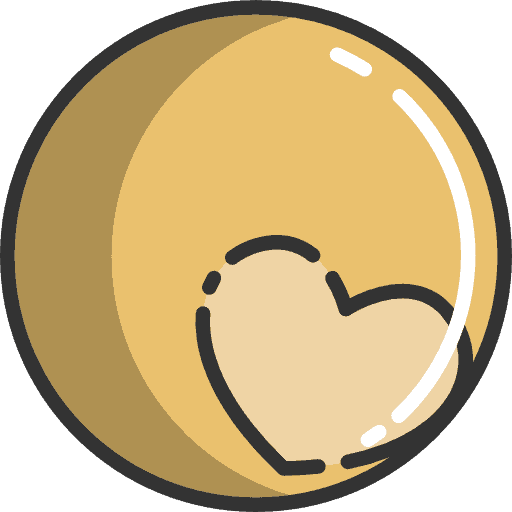
The Nine Planets
How Big is the Universe?
Think about this for a second; it takes us around three days to reach the Moon , approximately seven months to get the closest planet to us, namely Mars , 15 months to reach Venus , six years to reach Jupiter , seven to reach Saturn , 8.5 years to reach Uranus , 9.5 years to reach Pluto – the closest dwarf planet , and twelve years to get to Neptune , the farthest planet.
The Sun is 0.00001581 light-years away, and in the best-case scenario, we could reach it in 25 days. So how big is the Universe ? Its around 93 billion light-years. How much is that? Well, let’s think about the Sun again.

The Sun is one astronomical unit (AU) away from us. One astronomical unit is 149,598,000 km / 92,955,887 miles, and in our top shape, we could reach it in 25 days. Now, the Universe is 93 billion light-years across, and one, just one light-year, is equivalent to 63,000 astronomical units.
As such, one light-year is the equivalent to 9 trillion kilometers / 6 trillion miles, and our Universe is 93 billion light-years in diameter. That’s how big our Universe is, and that’s not even the end of it. The 93 billion years is just the observable Universe, the Universe, which we can currently see. The whole Universe might very well be 250 times larger than the observable Universe, or at least 7 trillion light-years in diameter.
Why is the Universe so Big?
The Universe is so big because it is constantly expanding, and it does so at a speed that even exceeds the speed of light. Space itself is actually growing, and this is going on for around 14 billion years or so.
In this amount of time, with speed greater than the speed of light, the Universe gradually grew, and it still expands even to this day. There isn’t actually an answer to why the Universe is this big.
Think about how big you are compared to an ant or an atom. There is a huge difference even there. It all has to do with our perception, and even in our current modern age, our perception of the Universe is vastly limited.

Think of it this way, we might see the Universe as being big now, but in the far future, who knows how future generations would view it. Our ancestors didn’t have cars or planes, and they would traverse the world in many months, even years. For them, traveling from point a to b seemed incredibly difficult, and they themselves might have thought, why is the Earth so big?
Nowadays, the world doesn’t seem so big anymore. You can reach point b from point a in a couple of minutes, hours, or in the worst case, days. Our perception is the only thing at play here.
In the future, who knows, maybe we could actually invent something that could travel at the speed of light. Perhaps we might even invent teleportation, or we could use wormholes.
If such technologies are there for us to grab, then will the Universe truly seem so big then? Perhaps it will, but if the vast distances which we should traverse are more easily done, and if the man of the future will find a way to prolong his life further, then the Universe will certainly begin to feel much smaller. Again, perception is everything at play here. We are asking the wrong questions; nature is what it is.
Is the Universe Really Infinite?
Many believe that our Universe is just 13.8 billion years old. However, this is uncertain until proven with extreme accuracy. Sometimes, we can’t even pinpoint the certain age of an object here on Earth, let alone our Universe.
The Universe may be infinite, or it might not be, but again, our perception is at play here. If we will analyze how many stars , planets, and the distances involved for reaching them, and the fact that our Universe is expanding, then it certainly seems infinite.
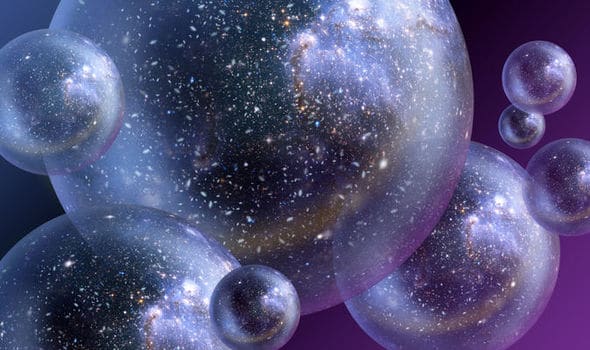
Even if our Universe weren’t infinite, we would view it as such due to its vastness, and the time it would take to explore everything in it. Our perception creates endless problems in the end.
Now, as you observe, our world is created from dualistic elements—day and night, hot or cold, love or hate, etc. Everything in our little world seems finite, so why shouldn’t there be an infinite element, such as our Universe? Many are afraid to admit that something is infinite, but no matter how you look at it, even if you would reach the end of the Universe, it would still seem infinite regardless.
How Big is the Universe Compared to the Observable Universe?
The observable Universe is 93 billion light-years in diameter. Some scientists believe its true size is even scarier than that. By using the Bayesian model averaging, scientists estimated that the Universe is at least 250 times larger than the observable Universe, or at least 7 trillion light-years in diameter.

The Bayesian model focuses on how likely a model is to be correct, given the data, rather than asking how well the model itself fits the data. Now, this might not be the best method of estimating the true size of our Universe. Still, chances are very high that our Universe is nonetheless, bigger than the observable Universe.
Does the Universe End?
Many cosmologists agree that the Universe is flat and might expand forever. Others theorize that the Universe will end one day. Perhaps the most logical answer is that when all the stars reach the end of their life spans, the Universe will end as the light will end, everything will be covered in darkness.
Maybe the Universe won’t end there if other means of survival are available. Some believe that the Universe will begin to cool as it expands, and life will cease to exist because of this. This theory is named the Big Freeze.
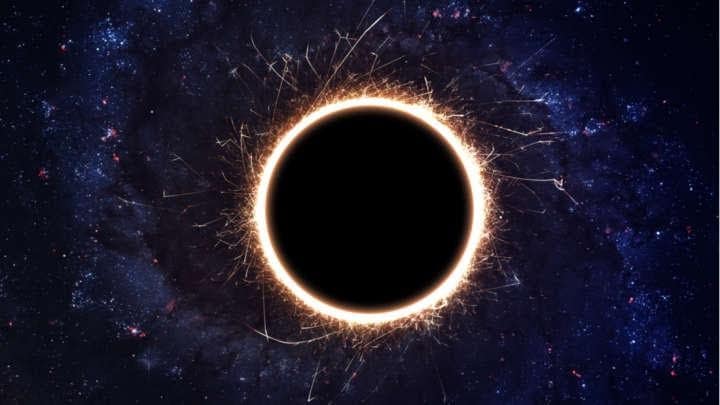
Another popular scenario suggests that our Universe will stop expanding, and will actually reverse this process. When this happens, the Universe will re-collapse, and it will possibly lead to a reformation that will start with another Big Bang. This scenario is called the Big Crunch.
A third popular theory is named the Big Rip. This theory states that everything will be torn to shreds, including atoms. It will happen when the theoretical energy known as dark energy, becomes stronger than gravity. No matter the case, what is certain is that these apocalyptic scenarios won’t happen for billions of years to come.
Where Does Space End?
Space doesn’t end because it is constantly expanding, faster than the speed of light itself. From our point of view, space end at approximately 93 billion light-years away from us, but that isn’t the end of the Universe. It is only how far we can see.
Will the Universe Last Forever?
It is unknown if the Universe will last forever, but most likely, we won’t even be there to see it. We currently do not know if the Univers will stop expanding, and if it does, what would this imply.
Many have proposed several apocalyptic scenarios, like the ones aforementioned above, but isn’t that just typical of us? The Universe might just well last forever, but one thing is true. We are very far from answering such questions.

Everything in the Universe is in motion, and it appears that many celestial objects, such as galaxies , are moving away from us. Perhaps, this is the true end of the Universe, when things will be so far apart that nothing could be reached anymore, and nothing could be concluded as being the Universe anymore since everything will be so far apart, we wouldn’t even know its there.
Did you Know?
- Around 550 people have been into Space, and only three of them have died in accidents.
- The smallest thing in the Universe that we currently know of is the atom.
- The biggest thing we have discovered so far in our Universe is the Hercules-Corona Borealis Great Wall. It is a supercluster which has a diameter of around 10 billion light-years.
- Many believe that our Universe is only one of a set of separate universes, collectively known as the multiverse.
- The word cosmos, rather than Universe, implies viewing the Universe as a complex and orderly system or deity – the opposite of chaos.
- The observable Universe is 93 billion light-years, yet, our galaxy, the Milky Way , is just 100,000 light-years in diameter. It would take us endless generations just to explore our galaxy, let alone the Universe.
- Another ancient structure is the galaxy supercluster known as the Hyperion Supercluster. This celestial object has more than four quadrillion solar masses, and many estimate that it formed just 2 billion years after the Big Bang.
- Universe means “whole,” and it comes from the Latin word “universus.”
- Livescience
- Scaleofuniverse
Image Sources:
- https://miro.medium.com/max/670/1*73_LKv_ioTH3TF5Hc0LFYg.jpeg
- https://thumbor.forbes.com/thumbor/960×0/https%3A%2F%2Fblogs-images.forbes.com%2Fstartswithabang%2Ffiles%2F2016%2F07%2F060915_CMB_Timeline150-1200×819.jpg
- https://cdn.images.express.co.uk/img/dynamic/151/590x/1-695868.jpg
- https://thumbor.forbes.com/thumbor/960×0/https%3A%2F%2Fblogs-images.forbes.com%2Fstartswithabang%2Ffiles%2F2017%2F05%2FSDSS_Deep.jpg
- https://cdn.iflscience.com/images/4a22cda7-0ef5-5226-9ed3-e80c4cf053c7/default-1510334289-cover-image.jpg
- https://images.theconversation.com/files/93501/original/image-20150901-25717-1y3igv.jpg?ixlib=rb-1.1.0&q=45&auto=format&w=496&fit=clip
How big is the universe?
How big is the universe around us? What we can observe gives us an answer, but it's likely much bigger than that.
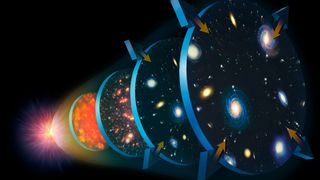
The observable universe
Measuring the universe, the shape of the universe, additional resources and reading, bibliography.
How big is the universe ? It's one of the fundamental questions of astronomy . By looking for the farthest observable point from Earth (and by extension, the oldest given the speed of light ) we can estimate a diameter.
Thanks to evolving technology, astronomers are able to look back in time to the moments just after the Big Bang . This might seem to imply that the entire universe lies within our view. But the size of the universe depends on a number of things, including its shape and expansion.
As a result, while we can make estimates as to the size of the universe scientists can't put a number on it.
Related: What is the coldest place in the universe?
In 2013, the European Space Agency's Planck space mission released the most accurate and detailed map ever made of the universe's oldest light. The map revealed that the universe is 13.8 billion years old. Planck calculated the age by studying the cosmic microwave background .
"The cosmic microwave background light is a traveler from far away and long ago," said Charles Lawrence, the U.S. project scientist for the mission at NASA's Jet Propulsion Laboratory in Pasadena, California, in a statement . "When it arrives, it tells us about the whole history of our universe."
Because of the connection between distance and the speed of light, this means scientists can look at a region of space that lies 13.8 billion light-years away. Like a ship in the empty ocean, astronomers on Earth can turn their telescopes to peer 13.8 billion light-years in every direction, which puts Earth inside of an observable sphere with a radius of 13.8 billion light-years. The word "observable" is key; the sphere limits what scientists can see but not what is there.
But though the sphere appears almost 28 billion light-years in diameter, it is far larger. Scientists know that the universe is expanding. Thus, while scientists might see a spot that lay 13.8 billion light-years from Earth at the time of the Big Bang, the universe has continued to expand over its lifetime. If inflation occurred at a constant rate through the life of the universe, that same spot is 46 billion light-years away today according to Ethan Siegel, writing for Forbes , making the diameter of the observable universe a sphere around 92 billion light-years.
These estimations are further complicated by the possibility that the universe is not expanding in an even manner. ESA reported on a 2020 study using data from ESA’s XMM-Newton, NASA’s Chandra Space Telescope and Rosat X-ray observatories suggests that the universe is not expanding at the same rate in all directions. The study measured the X-ray temperatures of hundreds of galaxy clusters and compared that against their brightness. Some clusters appeared less bright than expected, suggesting they were not moving at the same rate. "This possibly uneven effect on cosmic expansion might be caused by the mysterious dark energy ," ESA stated.
Centering a sphere on Earth's location in space might seem to put humans in the center of the universe. However, like that same ship in the ocean, we cannot tell where we lie in the enormous span of the universe. Just because we cannot see land does not mean we are in the center of the ocean; just because we cannot see the edge of the universe does not mean we lie in the center of the universe.
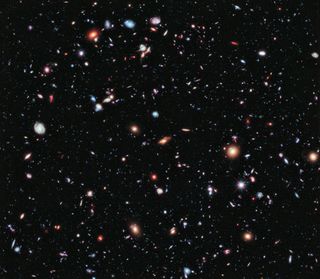
Scientists measure the size of the universe in a myriad of different ways. They can measure the waves from the early universe, known as baryonic acoustic oscillations, that fill the cosmic microwave background. They can also use standard candles, such as type 1A supernovae, to measure distances. However, these different methods of measuring distances can provide answers.
How inflation is changing is also a mystery. While the estimate of 92 billion light-years comes from the idea of a constant rate of inflation, many scientists think that the rate is slowing down. If the universe expanded at the speed of light during inflation, it should be 10^23, or 100 sextillion. One explanation for this, outlined by NASA in 2019, is that dark energy events may have impacted the expansion of the universe in the moments after the Big Bang.
Instead of taking one measurement method, a team of scientists led by Mihran Vardanyan at the University of Oxford did a statistical analysis of all of the results. By using Bayesian model averaging, which focuses on how likely a model is to be correct given the data, rather than asking how well the model itself fits the data. They found that the universe is at least 250 times larger than the observable universe, or at least 7 trillion light-years across.
"That's big, but actually more tightly constrained that many other models," according to 2011 MIT Technology Review report.
Related stories:
– Phantom energy and dark gravity: Explaining the dark side of the universe
– Do parallel universes exist? We might live in a multiverse
– Geocentric model: The Earth-centered view of the universe
The size of the universe depends a great deal on its shape. Scientists have predicted the possibility that the universe might be closed like a sphere, infinite and negatively curved like a saddle, or flat and infinite.
A finite universe has a finite size that can be measured; this would be the case in a closed spherical universe. But an infinite universe has no size by definition.
According to NASA, scientists know that the universe is flat with only about a 0.4 percent margin of error (as of 2013). And that could change our understanding of just how big the universe is.
"This suggests that the universe is infinite in extent; however, since the universe has a finite age, we can only observe a finite volume of the universe," says NASA . "All we can truly conclude is that the universe is much larger than the volume we can directly observe."
Determining the shape of the universe presents further challenges thanks to the limits of our means of observation. "Like a hall of mirrors, the apparently endless universe might be deluding us. The cosmos could, in fact, be finite. The illusion of infinity would come about as light wrapped all the way around space, perhaps more than once—creating multiple images of each galaxy," according to the University of Oregon department of physics .
There are plenty more questions about the universe you might want to have answered, such as what if the universe didn't have a beginning ? If your thirst for universal knowledge needs more, then these 10 wild theories about the universe might get your mind racing as well.
"Planck Mission Explores the History of Our Universe" NASA Jet Propulsion Laboratory
"How Big Was The Universe At The Moment Of Its Creation?" Forbes
"The Universe Might Not Be Expanding At The Same Rate Everywhere" ESA
"Mystery of the Universe's Expansion Rate Widens With New Hubble Data" NASA
"Cosmos At Least 250x Bigger Than Visible Universe, Say Cosmologists" MIT Technology Review
"The Universe Is Flat — Now What?" Space.com
"Will the Universe expand forever?" NASA
"Geometry of the Universe" University of Oregon department of physics
Join our Space Forums to keep talking space on the latest missions, night sky and more! And if you have a news tip, correction or comment, let us know at: [email protected].
Get the Space.com Newsletter
Breaking space news, the latest updates on rocket launches, skywatching events and more!
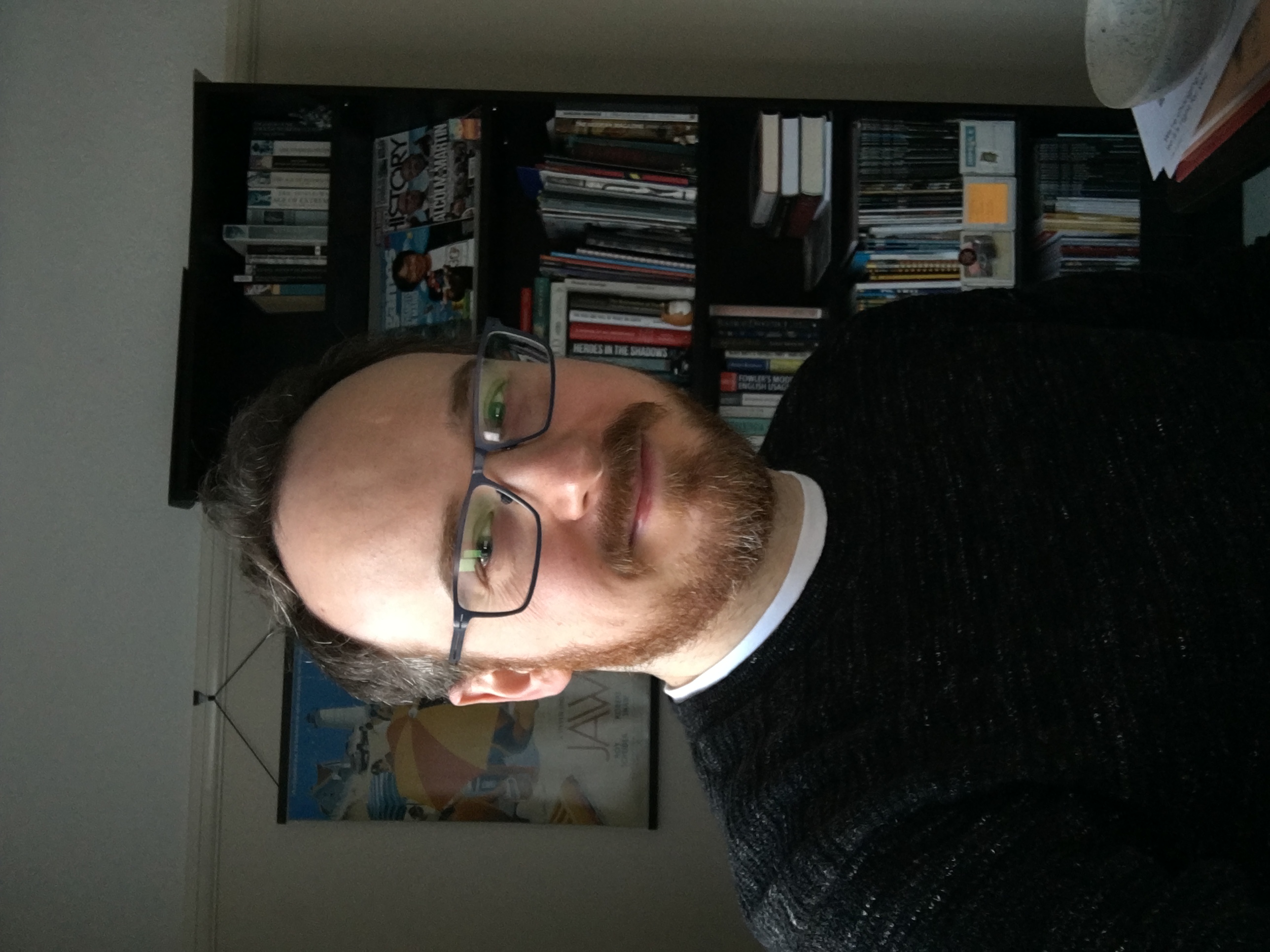
Jonathan is the Editor of All About History magazine. He has a degree in History from the University of Leeds. He has previously worked as editor of video game magazines games™ and X-ONE and tech magazines iCreate and Apps. He is currently based in Bournemouth, UK.
Science and music festival Starmus VII is about to rock Bratislava with a stellar lineup
China's Chang'e 6 mission to collect samples of the far side of the moon enters lunar orbit (video)
Jupiter's mysterious moon Amalthea spied crossing the Great Red Spot (photo)
Most Popular
- 2 Helium leak delays 1st astronaut launch of Boeing's Starliner to May 21
- 3 Giant 'rogue waves' of invisible matter might be disrupting the orbits of stars, new study hints
- 4 The summer of 2023 was Earth's hottest in 2,000 years, scientists find
- 5 SpaceX launching 50th mission of the year today
ASTR110G
Lecture slides for the entire course.
- Learning Objectives
- Self-Review Library
- 9. Spectrum Analysis -->
- Post-Class Survey
Copyright © 2011-2024 Nicole P. Vogt. All rights reserved.
Astronomy Picture of the Day
The Size of the Universe and Where Will We Go?
Discipline: Environmental Control/Life Support As an avid engineer and amateur astronomer, Mike Lawson presented a perspective on the size of the universe and asked the question, “Where will we go?” This was an entry-level overview for the average space worker who really wanted to understand the size of stars and the distance between objects in space. Mike provided information about familiar orbital objects and elaborated more on galaxies during the discussion. He also explored where humans could go in space and the physical limitations of going there.
Presenters:
- Confirmation of Attendance
- Mike Lawson's Biography
- NASA U.S. Spacesuit Knowledge Capture Website
- U.S. Spacesuit Knowledge Capture Program Catalog
- NESC Academy (Environmental Control/Life Support Catalog)
- NESC Academy Online
- Environmental Control/Life Support Community of Practice
Similar Videos
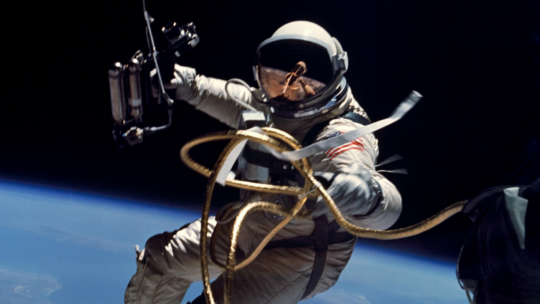
Stories and More
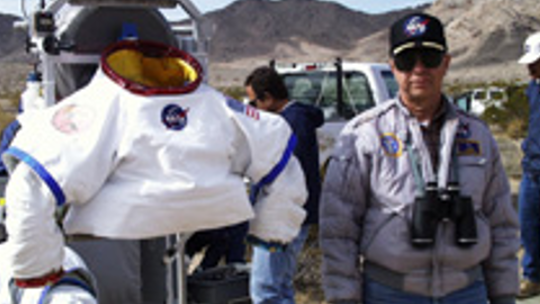
Kosmo's Farewell Advice
- The Universe
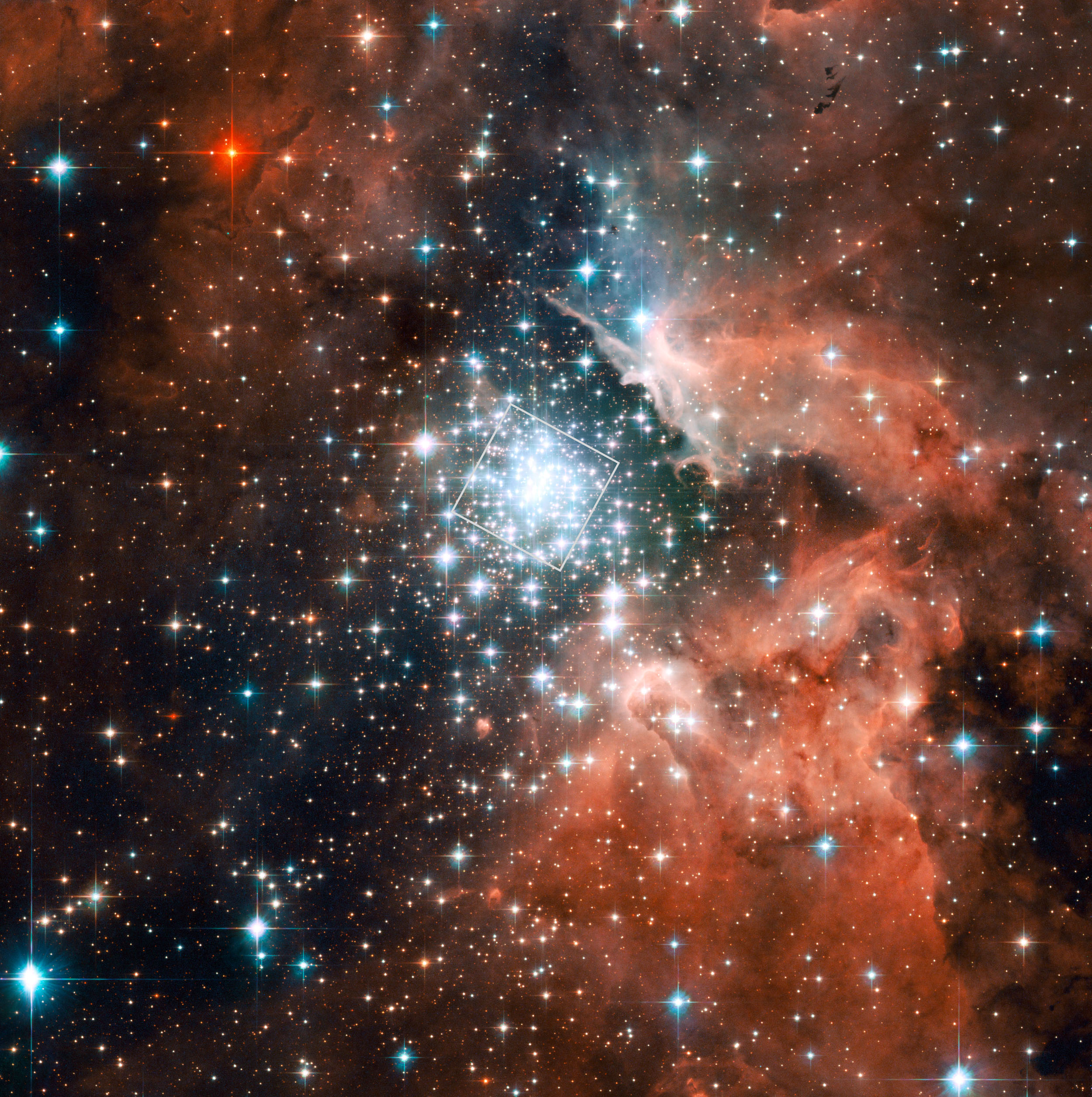
The Universe is everything we can touch, feel, sense, measure or detect. It includes living things, planets, stars, galaxies, dust clouds, light, and even time. Before the birth of the Universe, time, space and matter did not exist.
The Universe contains billions of galaxies, each containing millions or billions of stars. The space between the stars and galaxies is largely empty. However, even places far from stars and planets contain scattered particles of dust or a few hydrogen atoms per cubic centimeter. Space is also filled with radiation (e.g. light and heat), magnetic fields and high energy particles (e.g. cosmic rays).
The Universe is incredibly huge. It would take a modern jet fighter more than a million years to reach the nearest star to the Sun. Travelling at the speed of light (300,000 km per second), it would take 100,000 years to cross our Milky Way galaxy alone.
No one knows the exact size of the Universe, because we cannot see the edge – if there is one. All we do know is that the visible Universe is at least 93 billion light years across. (A light year is the distance light travels in one year – about 9 trillion km.)
The Universe has not always been the same size. Scientists believe it began in a Big Bang, which took place nearly 14 billion years ago. Since then, the Universe has been expanding outward at very high speed. So the area of space we now see is billions of times bigger than it was when the Universe was very young. The galaxies are also moving further apart as the space between them expands.
Story of the Universe
- Extreme life
- In the beginning
- The Big Bang
- The birth of galaxies
- What is space?
- Black Holes
- The mystery of the dark Universe
- Cosmic distances
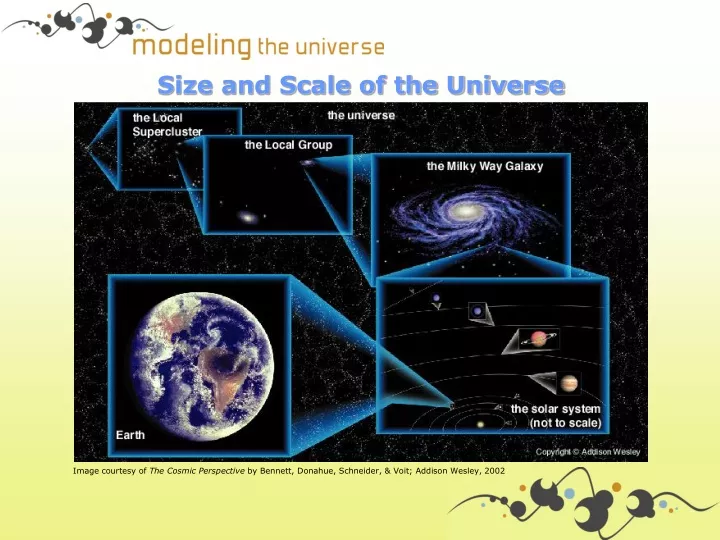
Size and Scale of the Universe
Jan 01, 2020
170 likes | 204 Views
Size and Scale of the Universe. Image courtesy of The Cosmic Perspective by Bennett, Donahue, Schneider, & Voit; Addison Wesley, 2002. Earth. Planet where we all live Comprised primarily of rock Spherical in shape 12,700 km in diameter
Share Presentation
- interstellar space
- average distance
- 390 light years
- 130 million light years
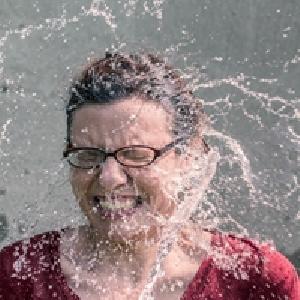
Presentation Transcript
Size and Scale of the Universe Image courtesy of The Cosmic Perspective by Bennett, Donahue, Schneider, & Voit; Addison Wesley, 2002
Earth • Planet where we all live • Comprised primarily of rock • Spherical in shape • 12,700 km in diameter • It would take 17 days to circumnavigate the globe driving a car at 100 km/hr • At the speed of light, it would take 0.13 seconds to go all the way around Earth.
Sun • Star that Earth orbits • Composed primarily of hydrogen and helium gas • Uses nuclear fusion in its core to generate heat and light to allow itself to resist the crushing weight of its own mass • Spherical in shape • 1.39 Million km in diameter
Earth & Sun • The Sun’s diameter is 109 times greater than that of Earth • Over 1 million Earths would fit inside the Sun’s volume • Earth orbits the Sun at an average distance of 150 million kilometers. This distance is called an Astronomical Unit (AU) • It would take 11,780 Earths lined up side to side to bridge the 1 AU between Earth and Sun.
The Solar System • 8.5 planets, thousands and thousands of planetoids and asteroids, billions of comets and meteoroids • Mostly distributed in a disk about the Sun • Sun blows a constant wind of charged gas into interplanetary space, called the Solar Wind Boundary between Solar Wind and interstellar space at 100 AU from the Sun (200 AU diameter)
The Solar Neighborhood • The region of the Galaxy within about 32.6 light-years of the Sun (65 light-years diameter) is considered its neighborhood. • Here stars move generally with the Sun in its orbit around the center of the Galaxy • This region is inside a large bubble of hot interstellar gas called the Local Bubble. Here the gas temperature is about 1 million degrees Kelvin and the density is 1000 times less than average interstellar space. To Center of Galaxy The image is 390 light-years across. Direction of Galactic Rotation
You Are Here The Milky Way Galaxy The Milky Way Galaxy is a giant disk of stars 160,000 light-years across and 1,000 light-years thick. The Sun is located at the edge of a spiral arm, 30,000 light-years from the center It takes 250 Million years for the Sun to complete one orbit There are over 100 Billion stars in the Milky Way The Spiral arms are only 5% more dense than average, and are the locations of new star formation
The Local Group • Contains 3 large spiral galaxies--Milky Way, Andromeda (M31), and Triangulum (M33)—plus a few dozen dwarf galaxies with elliptical or irregular shapes. • Gravitationally bound together—orbiting about a common center of mass • Ellipsoidal in shape • About 6.5 million light-years in diameter
A cluster of many groups and clusters of galaxies • Largest cluster is the Virgo cluster containing over a thousand galaxies. • Clusters and groups of galaxies are gravitationally bound together, however the clusters and groups spread away from each other as the Universe expands. • The Local Supercluster gets bigger with time • It has a flattened shape • The Local Group is on the edge of the majority of galaxies • The Local Supercluster is about 130 Million light-years across The Local Supercluster
1.3 Billion light-years The Universe • Surveys of galaxies reveal a web-like or honeycomb structure to the Universe • Great walls and filaments of matter surrounding voids containing no galaxies • Probably 100 Billion galaxies in the Universe The plane of the Milky Way Galaxy obscures our view of what lies beyond. This creates the wedge-shaped gaps in all-sky galaxy surveys such as those shown here.
The Universe The observable Universe is 27 Billion light-years in diameter. Computer Simulation
1) The Standard Ruler There are two basic methods for measuring astronomical distances • Use knowledge of physical and/or geometric properties of an object to relate an angular size with a physical size to determine distance. • Ex: Parallax, Moving Clusters, Time Delays, Water MASERs • Considered to be a direct or absolute measurement. R d d = R/Tan() R/
Requires very precise measurements of stellar positions, and long baselines Need telescopes with high resolution, and must observe over several years. Hipparchos satellite measured distances to tens of thousands of stars within 1,500 light-years of the Sun. Trigonometric Parallax
Use knowledge of physical and/or empirical properties of an object to determine its Luminosity, which yields distance via the Inverse Square Law of Light. Ex: Cepheid Variables, Supernovae, TRGB, Tully-Fisher Considered to be relative until tied to an absolute calibration. 2) The Standard Candle b = L/4d2
Cepheid Variable Stars There is a kind of giant star whose surface pulsates in and out with a regular period. That period of pulsation is related to the Luminosity of the star. LMC contains hundreds of known Cepheids all at the same distance. Which allows for robust determination of the Period Luminosity Relationship.
To measure cosmological distances a ladder of methods is used to reach further out into the Universe. Each “rung” in the ladder of distance measuring methods depends on the calibration of the methods “below.”
- More by User
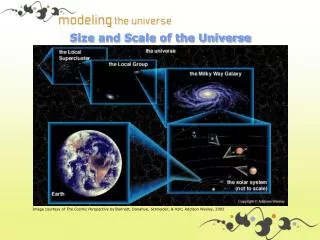
670 views • 16 slides
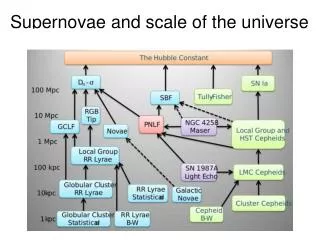
Supernovae and scale of the universe
Supernovae and scale of the universe. SN Ia have extremely uniform light curves → standard candles!. Supernovae and scale of the universe.
552 views • 38 slides
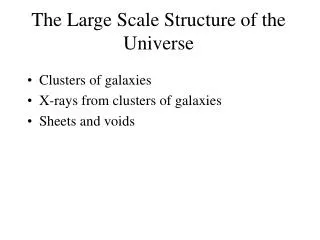
The Large Scale Structure of the Universe
The Large Scale Structure of the Universe. Clusters of galaxies X-rays from clusters of galaxies Sheets and voids. Our Galaxy is a member of a small cluster called the Local Group. Stephane’s Quintet. Virgo cluster. Abell 2218. Coma cluster. Virial Theorem.
352 views • 22 slides
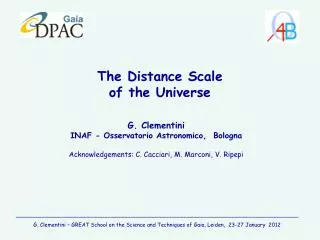
The Distance Scale of the Universe
The Distance Scale of the Universe. G. Clementini INAF - Osservatorio Astronomico , Bologna Acknowledgements: C. Cacciari , M. Marconi, V. Ripepi. __________________________________________________________________________________
789 views • 58 slides
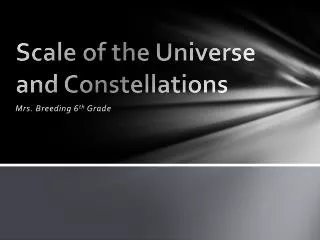
Scale of the Universe and Constellations
Scale of the Universe and Constellations. Mrs. Breeding 6 th Grade. Light Years. The fastest thing that we know of is light which travels at a speed of 186,000 miles per second in empty space .
263 views • 8 slides
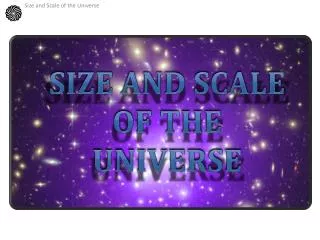
SIZE and Scale Of The Universe. Size and Scale of the Universe. Realms of the Universe. Size and Scale of the Universe. Image courtesy of The Cosmic Perspective by Bennett, Donahue, Schneider, & Voit ; Addison Wesley, 2002. Comprised primarily of rock Spherical in shape
683 views • 14 slides
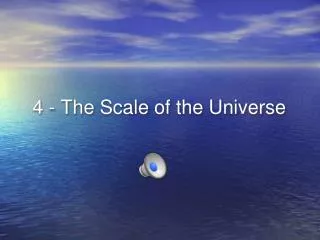
4 - The Scale of the Universe
4 - The Scale of the Universe. Distances - stellar parallax (sometimes called “ heliocentric parallax ” ). Click on me. http://www.astro.ubc.ca/~scharein/a311/Sim.html. pc ⇒ parsec = “ par allax sec ond ” (1 pc ~ 3.26 “ light-years ” ).
292 views • 8 slides
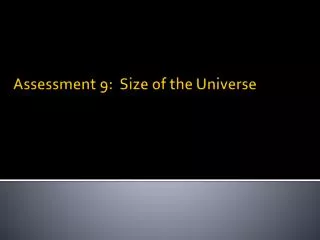
Assessment 9: Size of the Universe
Assessment 9: Size of the Universe. Measuring Distance. Scientific Notation: Use powers of 10 to write very large or very small numbers in shorter form Light Year : Light travels at the speed of 300,000,000 meters per second . That 300,000km a second!
238 views • 16 slides
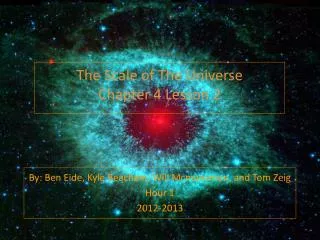
Scale of the Universe
The Scale of The Universe Chapter 4 Lesson 2. Scale of the Universe. By: Ben Eide, Kyle Beacham, Will Mcmanaman, and Tom Zeig Hour 1 2012-2013. How Do Astronomers Measure Distances to the Stars?. Vocab
316 views • 4 slides
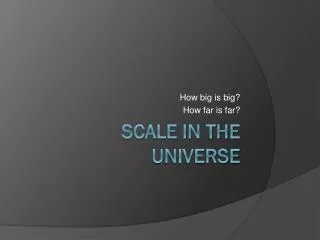
Scale In the Universe
How big is big? How far is far?. Scale In the Universe. What is a Million? . 1,000,000 seconds is equal to 11 days, 13 hours, 46 minutes, and 40 seconds. What is a Billion? . 1,000,000,000 (one Billion) seconds = 11, 500 days or 31.5 years!! One billion seconds ago, it was 1980.
305 views • 14 slides
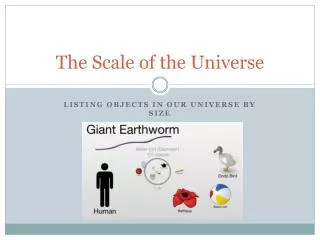
The Scale of the Universe
The Scale of the Universe. Listing objects in our Universe by size. The Scale of the Universe. Which object is the largest? Which object is the smallest?. Prediction. From the list given, list the objects from the smallest to the biggest What strategies can we use to sort the list?.
504 views • 7 slides
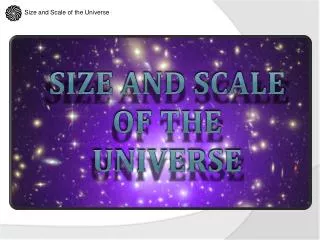
Size and Scale of the Universe. SIZE and Scale Of The Universe. Size and Scale of the Universe. # Street City State Country Continent Hemisphere. Planet Orbit Star? …? … … … …. What is your Cosmic Address?. Size and Scale of the Universe. Size and Scale of the Universe.
928 views • 22 slides
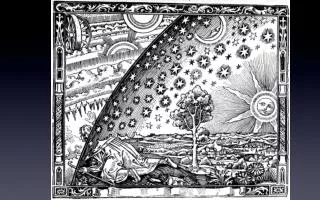
Fermi Problems and Scale of the Universe
Fermi Problems and Scale of the Universe. Advanced Introduction to Astronomy Spring 2014 Pomona College. by Dr. Bryan E. Penprase. An Example of a “ Flipped ” Class!!. Enrico Fermi, U. of Chicago Physics professor (and pioneer of nuclear physics) at the chalkboard. Fermi Problems.
399 views • 21 slides
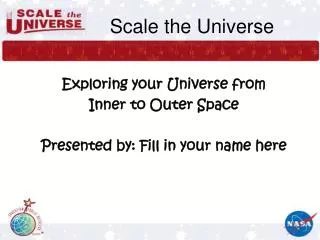
Scale the Universe
Scale the Universe. Exploring your Universe from Inner to Outer Space Presented by: Fill in your name here. The NASA Educator Ambassador Program at SSU. You should modify this slide to fit you. Swift. GLAST. XMM-Newton. What is GLAST?. GLAST: Gamma-Ray Large Area Space Telescope
551 views • 34 slides
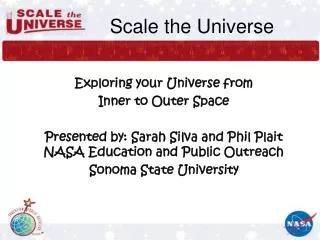
Scale the Universe. Exploring your Universe from Inner to Outer Space Presented by: Sarah Silva and Phil Plait NASA Education and Public Outreach Sonoma State University. The NASA E/PO Program at Sonoma State University.
240 views • 13 slides
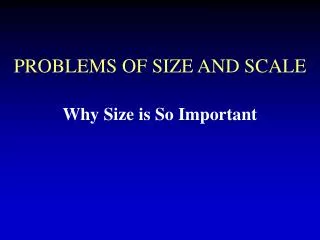
PROBLEMS OF SIZE AND SCALE
PROBLEMS OF SIZE AND SCALE. Why Size is So Important. We know that a whale. requires more energy (food, calories) per day than a mouse. Metabolic Rate vs. Body Size Scaling. But how much more?. written by Jonathan Swift in 1726. Gulliver in Lilliput - Lilliputian King is faced
207 views • 13 slides
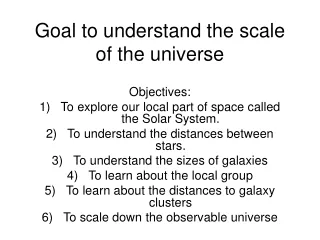
Goal to understand the scale of the universe
Goal to understand the scale of the universe. Objectives: To explore our local part of space called the Solar System. To understand the distances between stars. To understand the sizes of galaxies To learn about the local group To learn about the distances to galaxy clusters
246 views • 18 slides
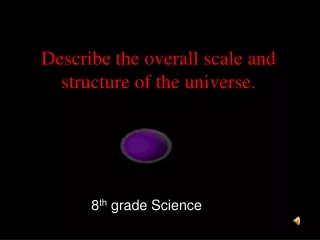
Describe the overall scale and structure of the universe.
Describe the overall scale and structure of the universe. 8 th grade Science.
151 views • 14 slides
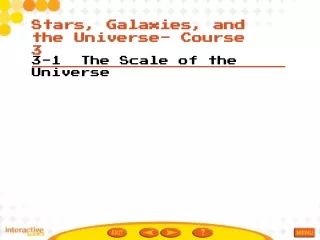
3-1 The Scale of the Universe
Stars, Galaxies, and the Universe- Course 3. 3-1 The Scale of the Universe. My Planet Diary pg. 94. Florida Space History Voyager Golden Record
120 views • 10 slides
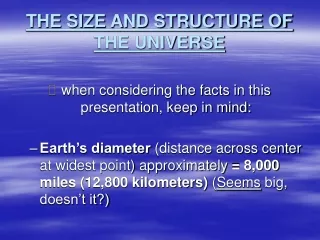
THE SIZE AND STRUCTURE OF THE UNIVERSE
THE SIZE AND STRUCTURE OF THE UNIVERSE. when considering the facts in this presentation, keep in mind: Earth’s diameter (distance across center at widest point) approximately = 8,000 miles (12,800 kilometers) ( Seems big, doesn’t it?). Meteors, Comets, Asteroids, Moons.
388 views • 24 slides
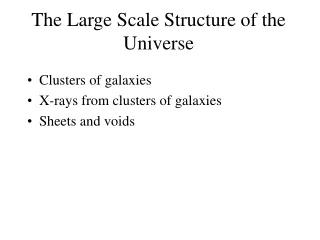
239 views • 22 slides
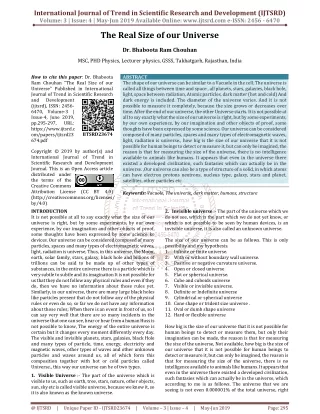
The Real Size of Our Universe
The shape of our universe can be similar to a Vacuole in the cell. The universe is called all things between time and space , all planets, stars, galaxies, black hole, light, space between radiation, Atomic particles, dark matter hot and cold And dark energy is included. The diameter of the universe varies. And it is not possible to measure it completely, because the size grows or decreases over time. After the end of our universe, the other Universe starts. It is not possible at all to say exactly what the size of our universe is right, but by some experiments, by our own experience, by our imagination and other objects of proof, some thoughts have been expressed by some science. Our universe can be considered composed of many particles, spaces and many types of electromagnetic waves, light, radiation is universe., how big is the size of our universe that it is not possible for human beings to detect or measure it, but can only be imagined, the reason is that for measuring the size of the universe, there is no intelligence available to animals like humans. It appears that even in the universe there existed a developed civilization, such fantasies which can actually be in the universe. ,Our universe can also be a type of structure of a solid, in which atoms can have electron protons neutrons, nucleus type, galaxy, stars and planet, satellites, other particles etc Dr. Bhaboota Ram Chouhan "The Real Size of our Universe" Published in International Journal of Trend in Scientific Research and Development (ijtsrd), ISSN: 2456-6470, Volume-3 | Issue-4 , June 2019, URL: https://www.ijtsrd.com/papers/ijtsrd23674.pdf Paper URL: https://www.ijtsrd.com/physics/astrophysics/23674/the-real-size-of-our-universe/dr-bhaboota-ram-chouhan
38 views • 3 slides
Got any suggestions?
We want to hear from you! Send us a message and help improve Slidesgo
Top searches
Trending searches
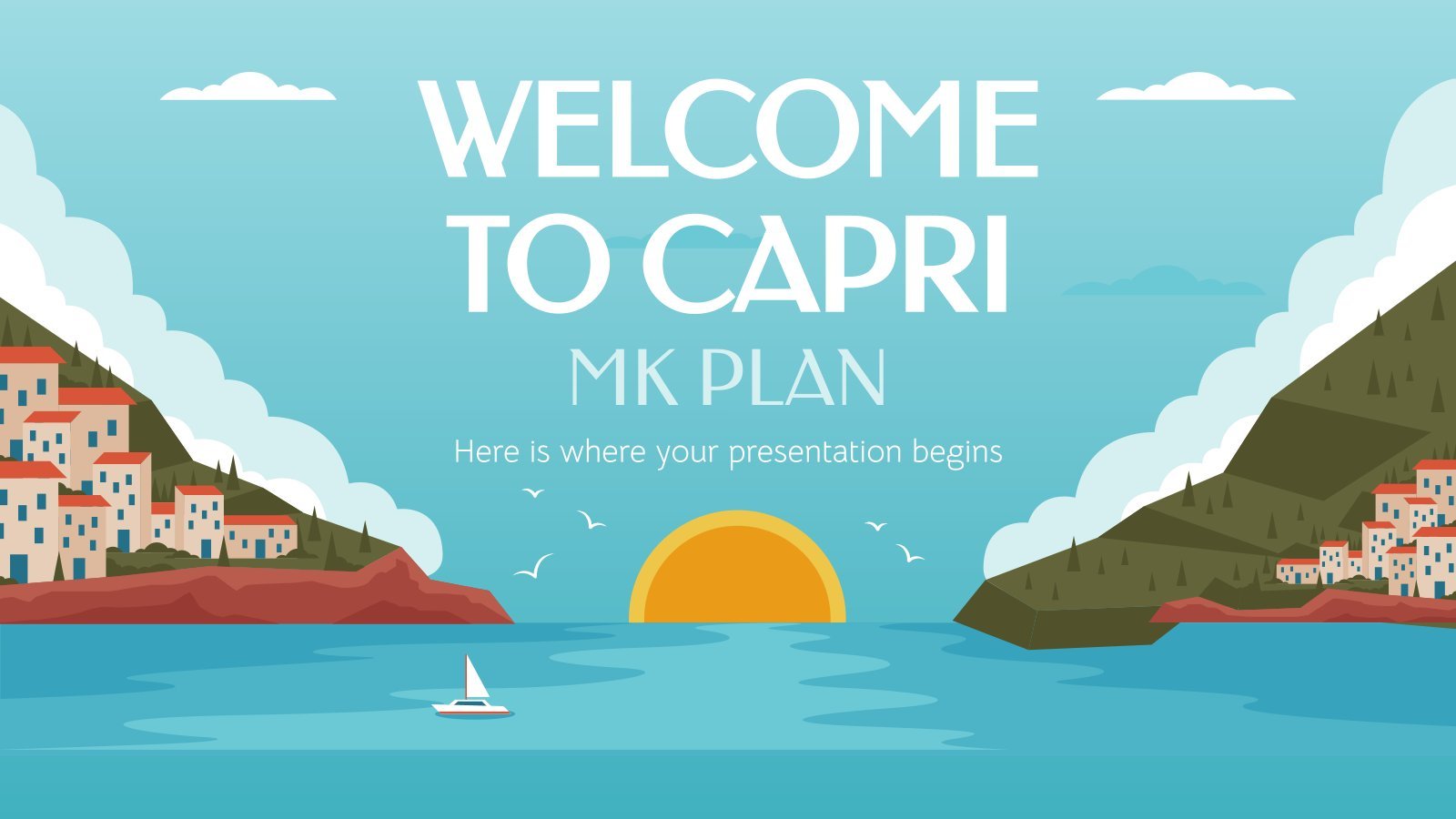
11 templates
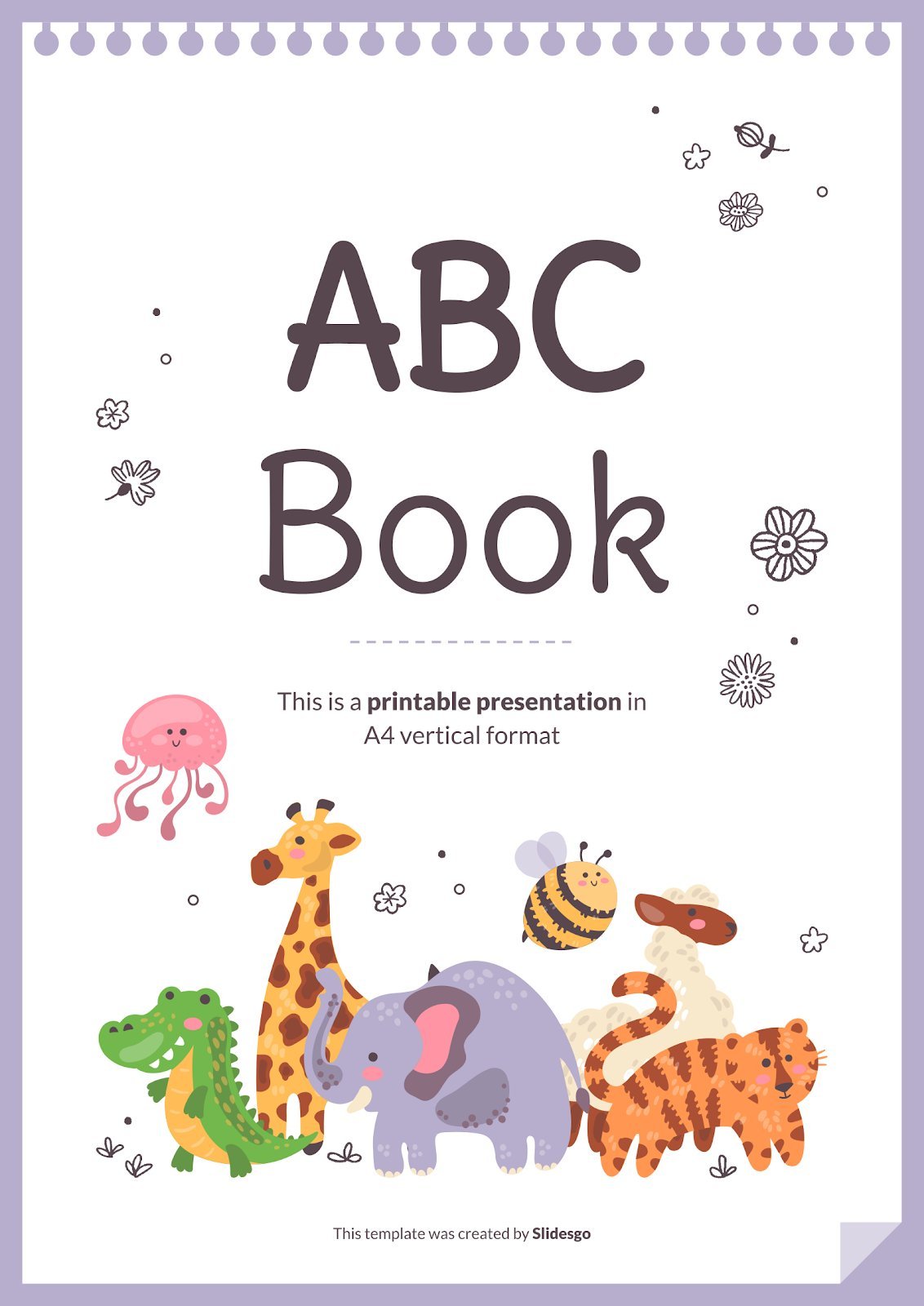
9 templates
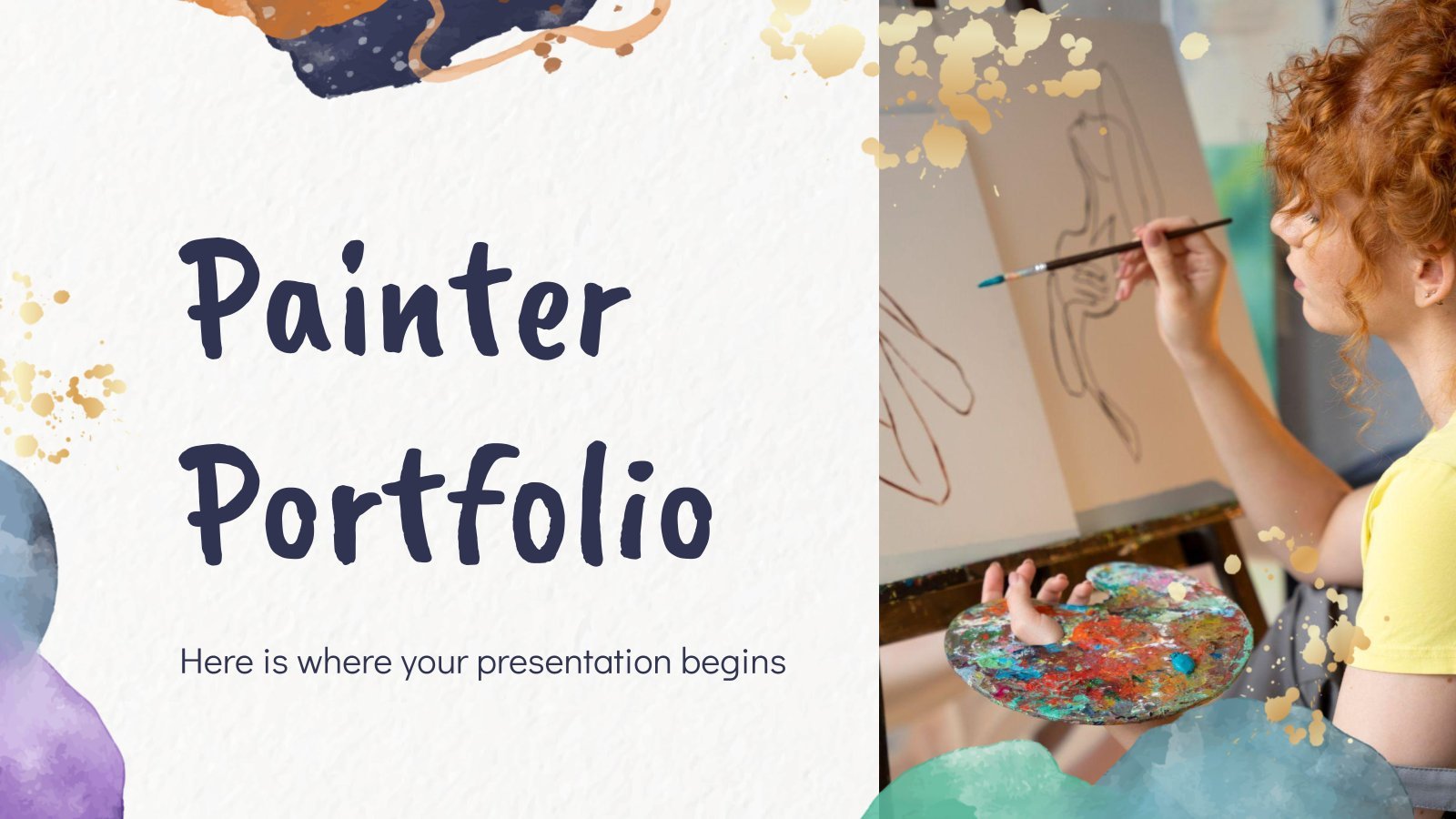
art portfolio
79 templates
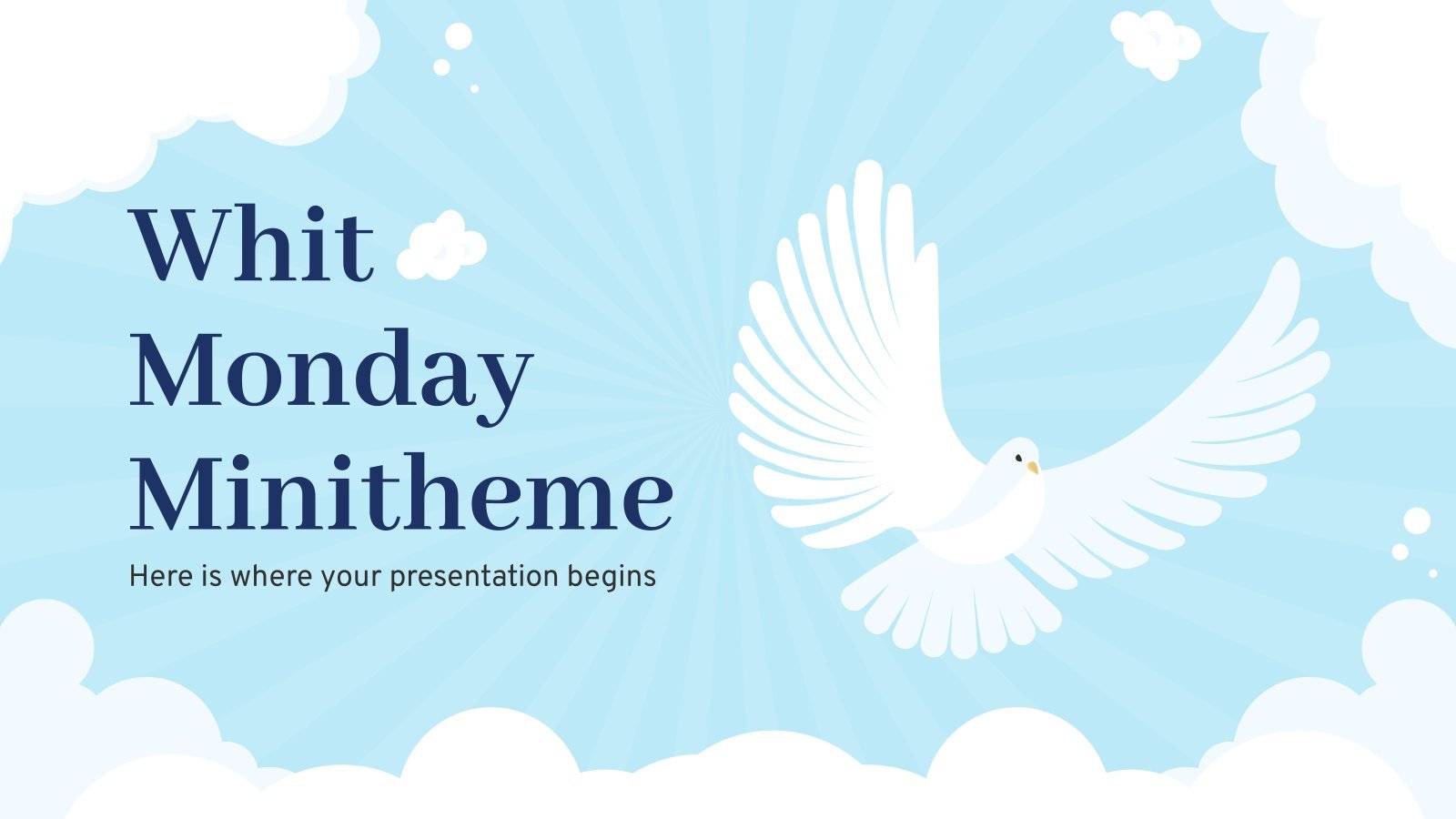
holy spirit
36 templates
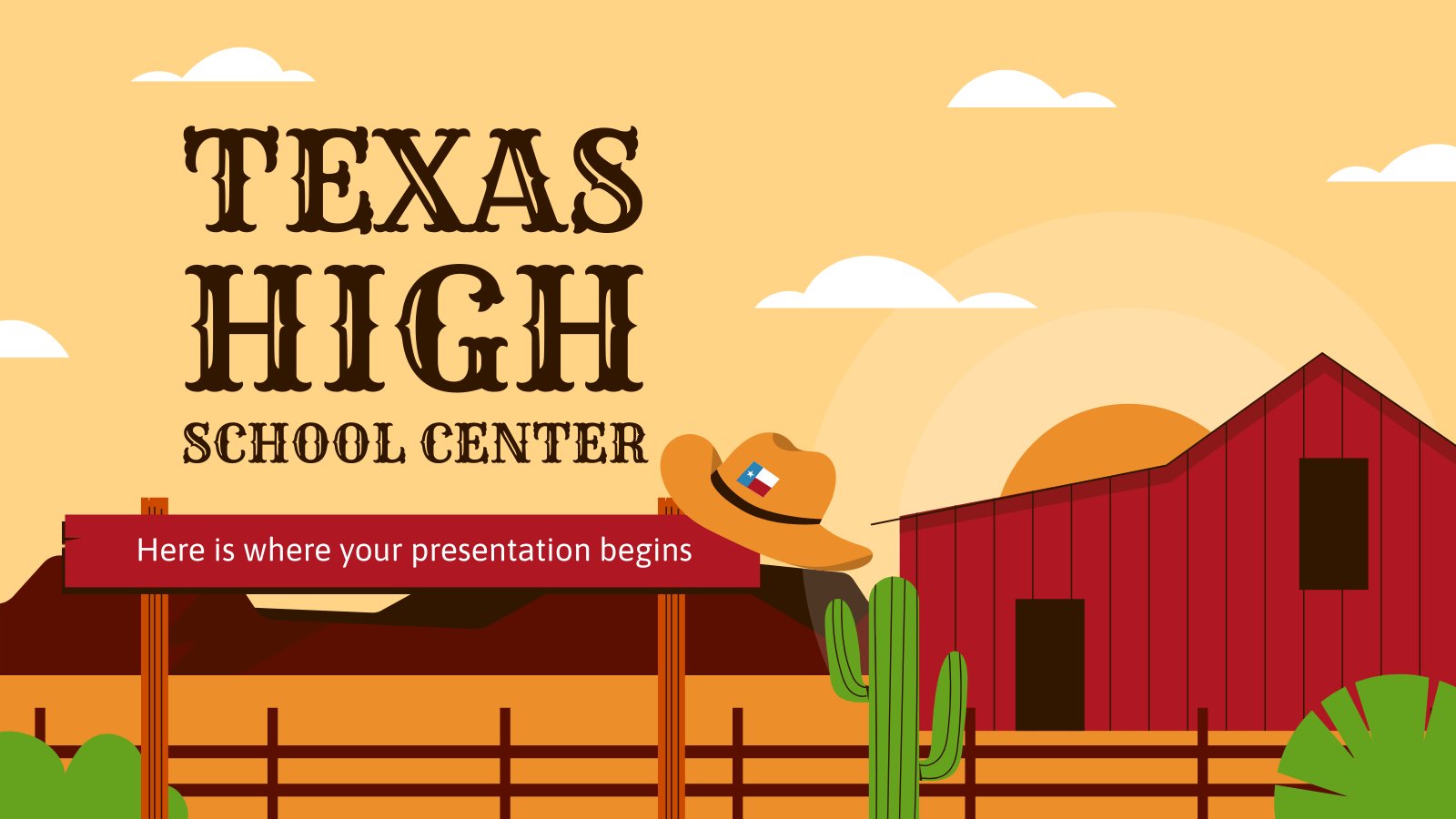
32 templates
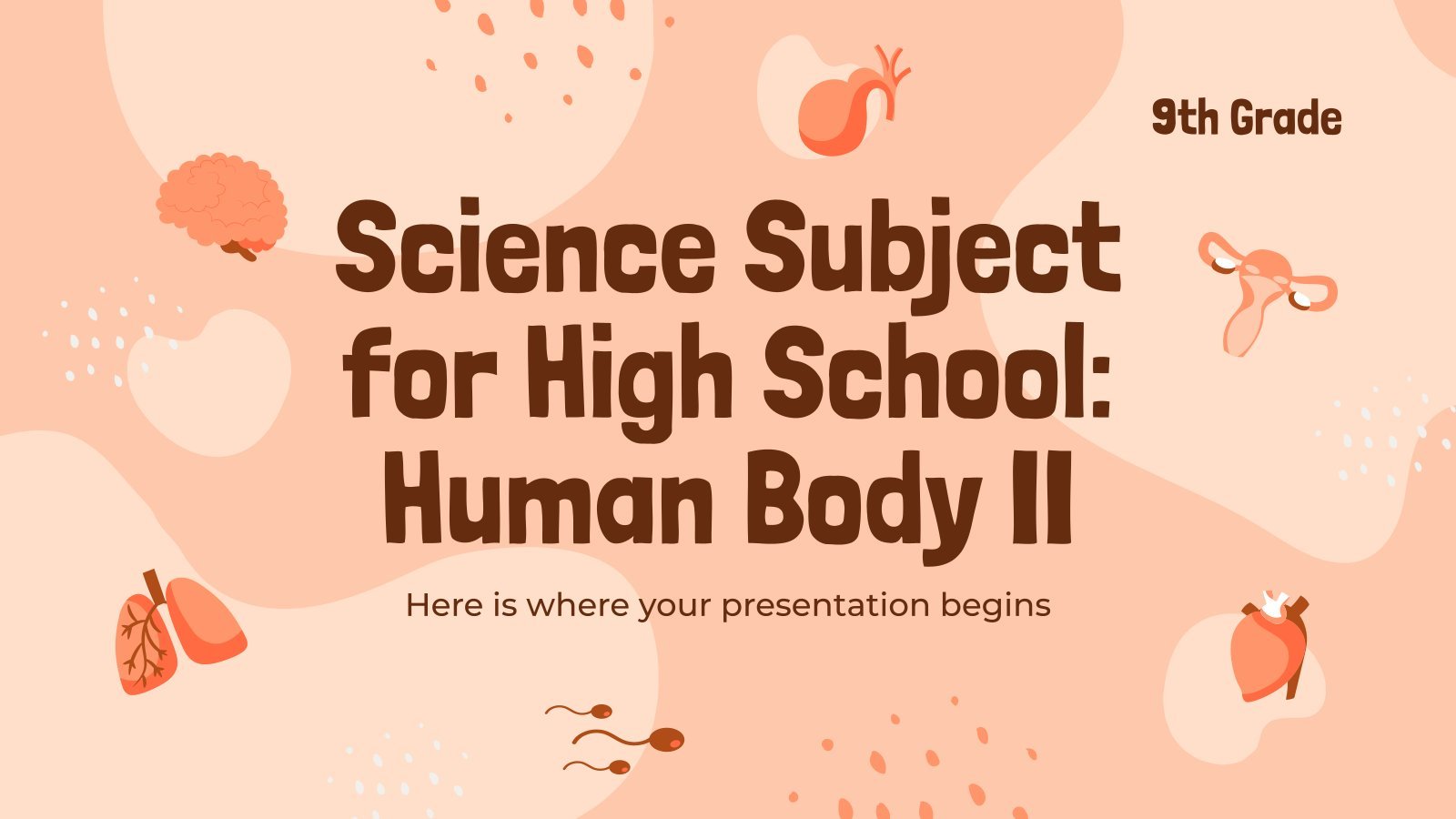
human anatomy
18 templates
Know The Universe: Astronomy Lesson
Know the universe: astronomy lesson presentation, free google slides theme, powerpoint template, and canva presentation template.
If you are an astronomy teacher you have to see this template. It is specially designed to create a fun presentation about this science. It has a clear background on which we have included a multitude of doodle-style illustrations that your audience will love. In addition, the typography of the titles is handwritten style, which also gives it an original touch. As for the color of the texts, blue and black are combined. And as for the resources included, there are tables, graphs, diagrams, timelines, etc.
Features of this template
- 100% editable and easy to modify
- 28 different slides to impress your audience
- Contains easy-to-edit graphics such as graphs, maps, tables, timelines and mockups
- Includes 500+ icons and Flaticon’s extension for customizing your slides
- Designed to be used in Google Slides, Canva, and Microsoft PowerPoint
- 16:9 widescreen format suitable for all types of screens
- Includes information about fonts, colors, and credits of the free resources used
How can I use the template?
Am I free to use the templates?
How to attribute?
Attribution required If you are a free user, you must attribute Slidesgo by keeping the slide where the credits appear. How to attribute?
Related posts on our blog.
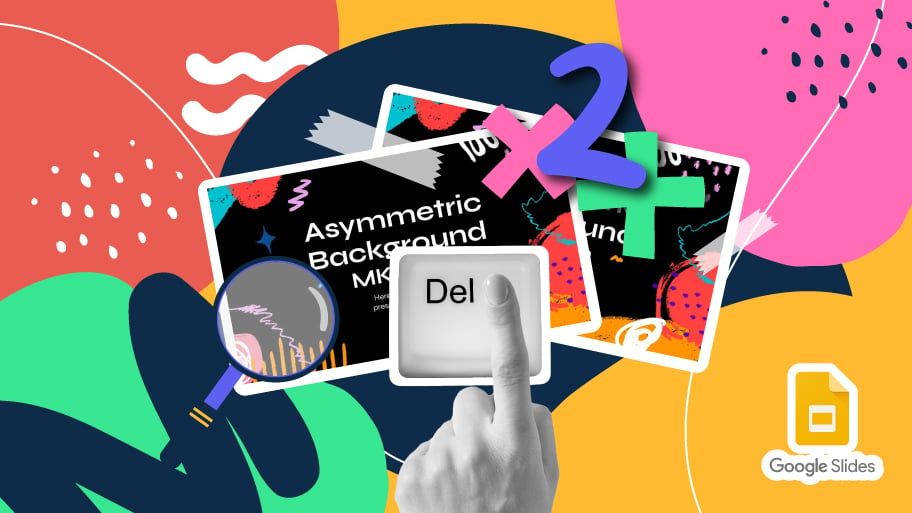
How to Add, Duplicate, Move, Delete or Hide Slides in Google Slides

How to Change Layouts in PowerPoint
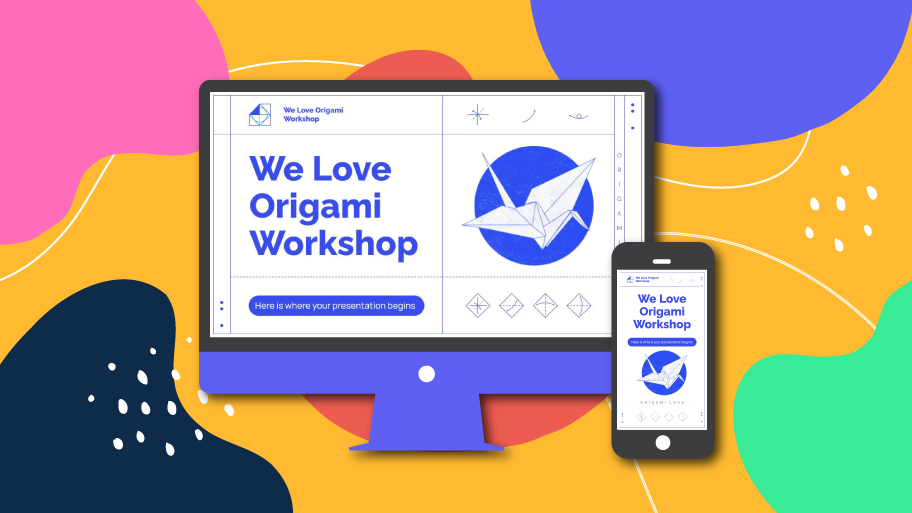
How to Change the Slide Size in Google Slides
Related presentations.
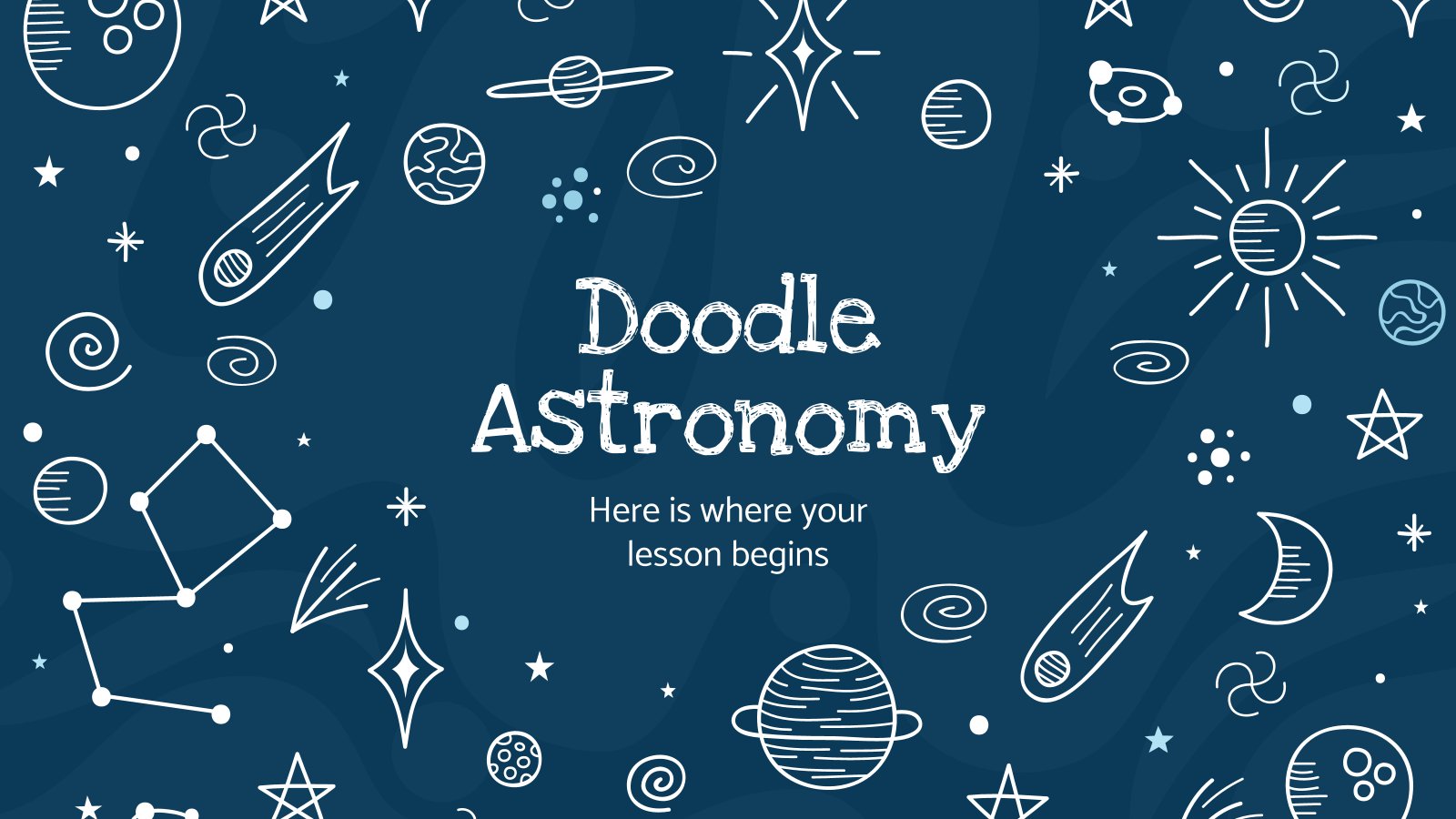
Premium template
Unlock this template and gain unlimited access
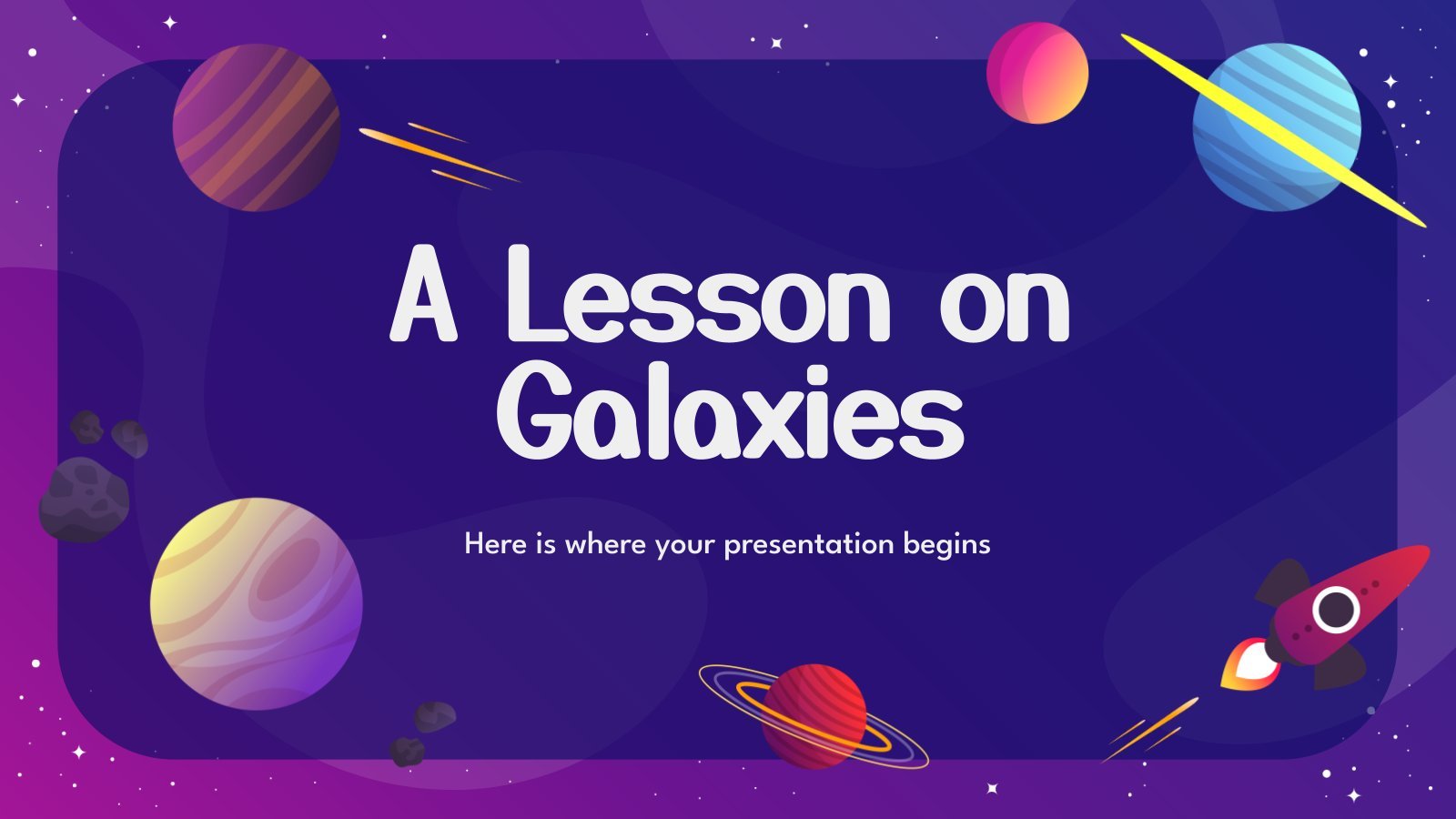
Origins of the universe, explained
The most popular theory of our universe's origin centers on a cosmic cataclysm unmatched in all of history—the big bang.
The best-supported theory of our universe's origin centers on an event known as the big bang. This theory was born of the observation that other galaxies are moving away from our own at great speed in all directions, as if they had all been propelled by an ancient explosive force.
A Belgian priest named Georges Lemaître first suggested the big bang theory in the 1920s, when he theorized that the universe began from a single primordial atom. The idea received major boosts from Edwin Hubble's observations that galaxies are speeding away from us in all directions, as well as from the 1960s discovery of cosmic microwave radiation—interpreted as echoes of the big bang—by Arno Penzias and Robert Wilson.
Further work has helped clarify the big bang's tempo. Here’s the theory: In the first 10^-43 seconds of its existence, the universe was very compact, less than a million billion billionth the size of a single atom. It's thought that at such an incomprehensibly dense, energetic state, the four fundamental forces—gravity, electromagnetism, and the strong and weak nuclear forces—were forged into a single force, but our current theories haven't yet figured out how a single, unified force would work. To pull this off, we'd need to know how gravity works on the subatomic scale, but we currently don't.
It's also thought that the extremely close quarters allowed the universe's very first particles to mix, mingle, and settle into roughly the same temperature. Then, in an unimaginably small fraction of a second, all that matter and energy expanded outward more or less evenly, with tiny variations provided by fluctuations on the quantum scale. That model of breakneck expansion, called inflation, may explain why the universe has such an even temperature and distribution of matter.
After inflation, the universe continued to expand but at a much slower rate. It's still unclear what exactly powered inflation.
Aftermath of cosmic inflation
As time passed and matter cooled, more diverse kinds of particles began to form, and they eventually condensed into the stars and galaxies of our present universe.
For Hungry Minds
By the time the universe was a billionth of a second old, the universe had cooled down enough for the four fundamental forces to separate from one another. The universe's fundamental particles also formed. It was still so hot, though, that these particles hadn't yet assembled into many of the subatomic particles we have today, such as the proton. As the universe kept expanding, this piping-hot primordial soup—called the quark-gluon plasma—continued to cool. Some particle colliders, such as CERN's Large Hadron Collider , are powerful enough to re-create the quark-gluon plasma.
Radiation in the early universe was so intense that colliding photons could form pairs of particles made of matter and antimatter, which is like regular matter in every way except with the opposite electrical charge. It's thought that the early universe contained equal amounts of matter and antimatter. But as the universe cooled, photons no longer packed enough punch to make matter-antimatter pairs. So like an extreme game of musical chairs, many particles of matter and antimatter paired off and annihilated one another.
You May Also Like
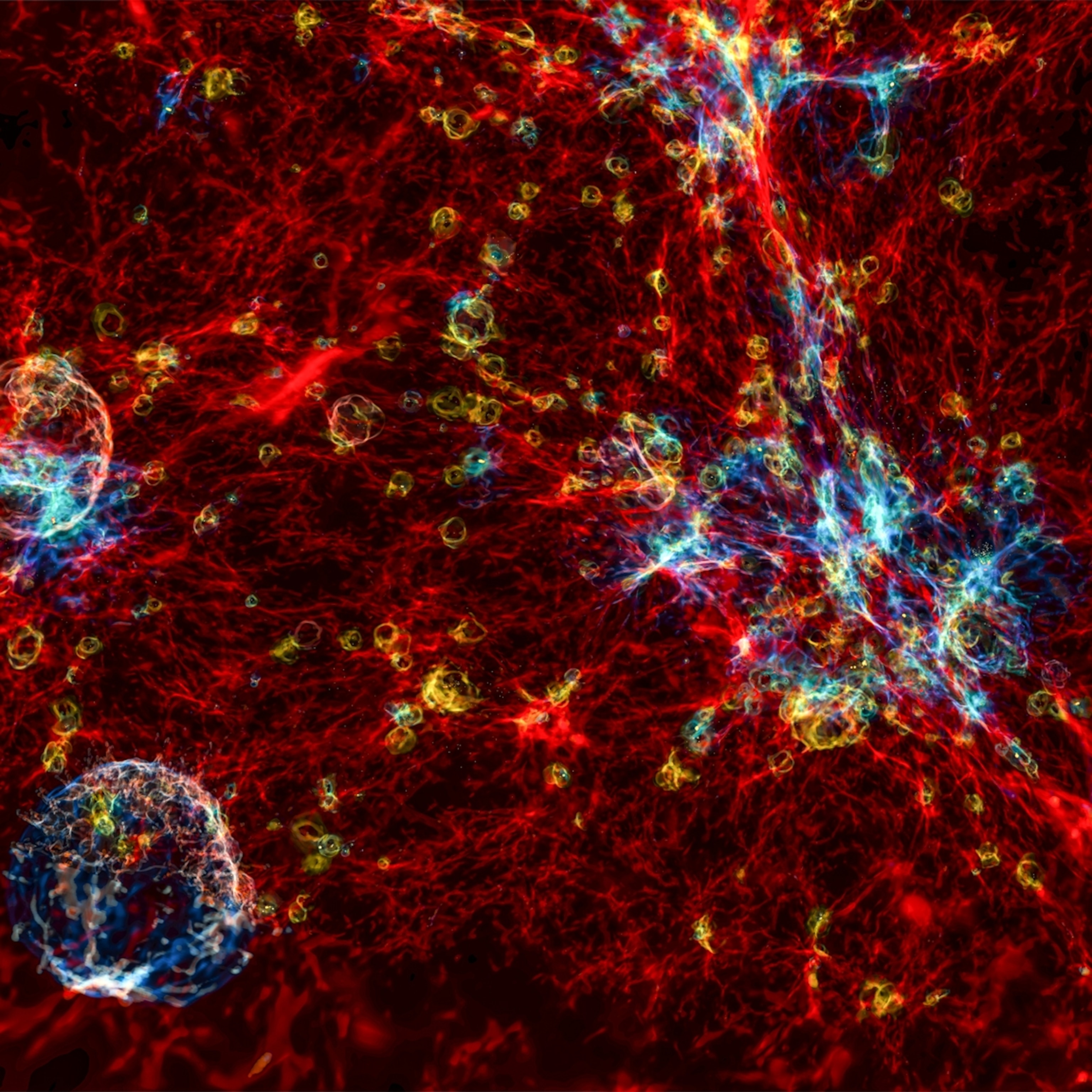
The world’s most powerful telescope is rewriting the story of space and time
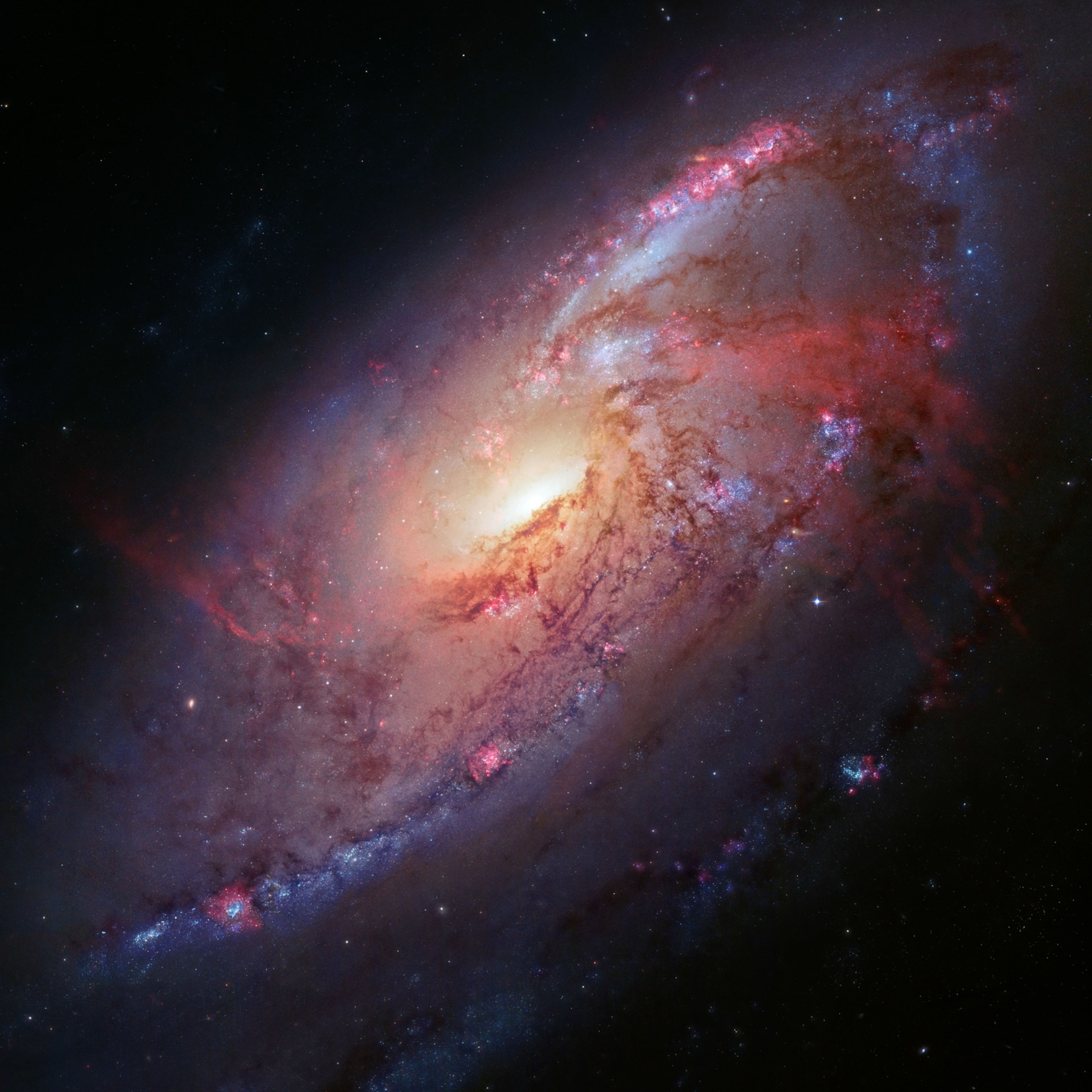
How fast is the universe really expanding? The mystery deepens.
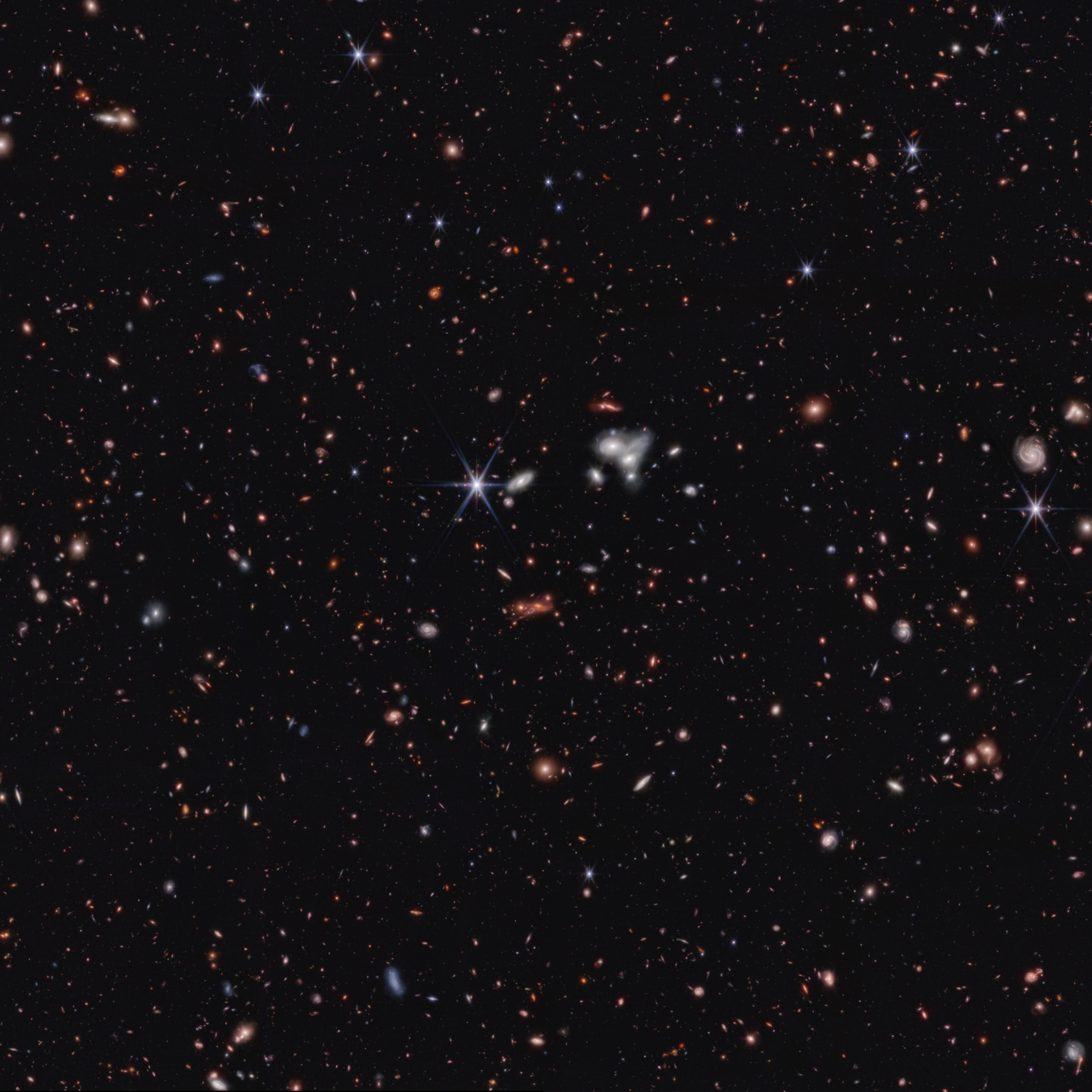
This supermassive black hole was formed when the universe was a toddler
Somehow, some excess matter survived—and it's now the stuff that people, planets, and galaxies are made of. Our existence is a clear sign that the laws of nature treat matter and antimatter slightly differently. Researchers have experimentally observed this rule imbalance, called CP violation , in action. Physicists are still trying to figure out exactly how matter won out in the early universe.
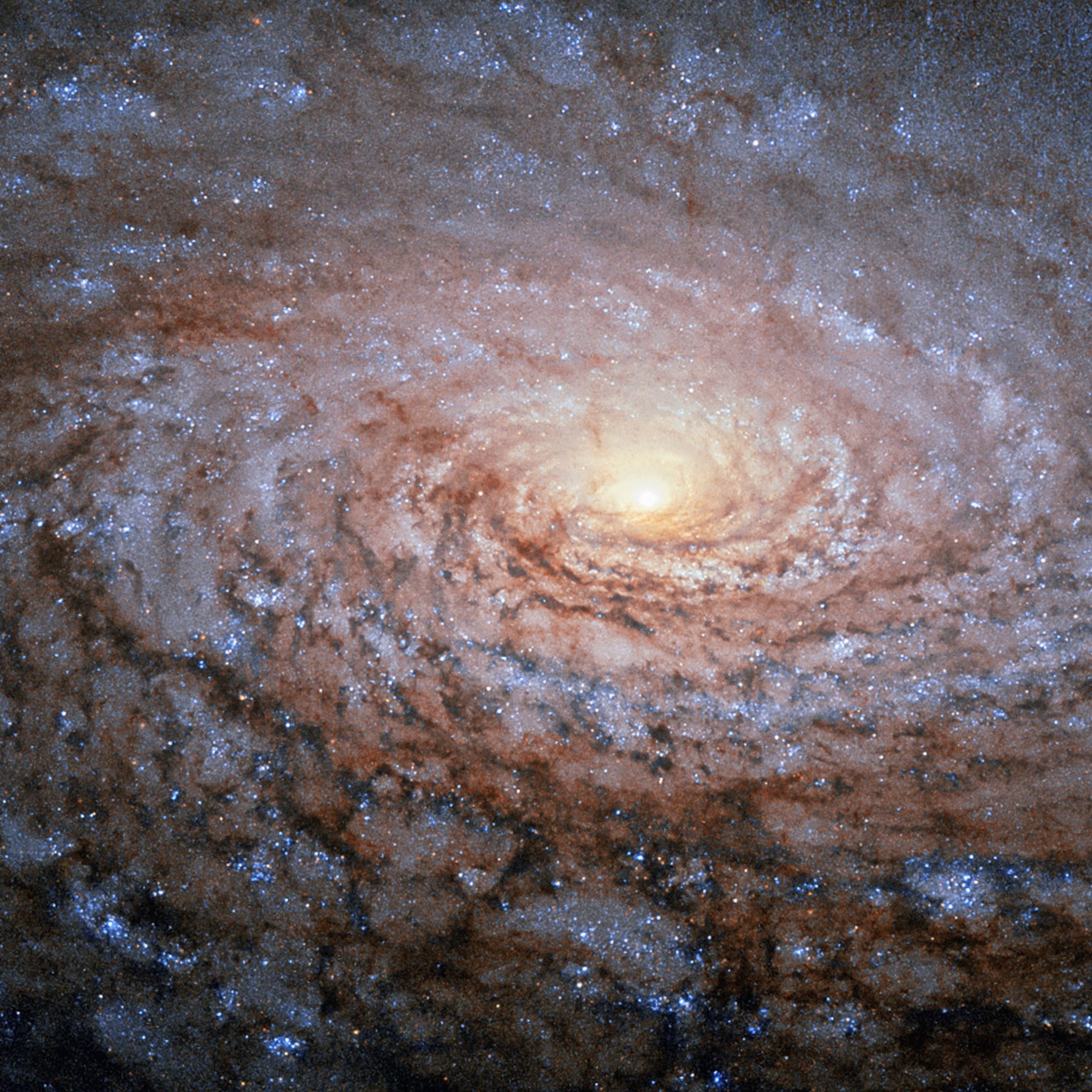
Building atoms
Within the universe's first second, it was cool enough for the remaining matter to coalesce into protons and neutrons, the familiar particles that make up atoms' nuclei. And after the first three minutes, the protons and neutrons had assembled into hydrogen and helium nuclei. By mass, hydrogen was 75 percent of the early universe's matter, and helium was 25 percent. The abundance of helium is a key prediction of big bang theory, and it's been confirmed by scientific observations.
Despite having atomic nuclei, the young universe was still too hot for electrons to settle in around them to form stable atoms. The universe's matter remained an electrically charged fog that was so dense, light had a hard time bouncing its way through. It would take another 380,000 years or so for the universe to cool down enough for neutral atoms to form—a pivotal moment called recombination. The cooler universe made it transparent for the first time, which let the photons rattling around within it finally zip through unimpeded.
We still see this primordial afterglow today as cosmic microwave background radiation , which is found throughout the universe. The radiation is similar to that used to transmit TV signals via antennae. But it is the oldest radiation known and may hold many secrets about the universe's earliest moments.
From the first stars to today
There wasn't a single star in the universe until about 180 million years after the big bang. It took that long for gravity to gather clouds of hydrogen and forge them into stars. Many physicists think that vast clouds of dark matter , a still-unknown material that outweighs visible matter by more than five to one, provided a gravitational scaffold for the first galaxies and stars.
Once the universe's first stars ignited , the light they unleashed packed enough punch to once again strip electrons from neutral atoms, a key chapter of the universe called reionization. In February 2018, an Australian team announced that they may have detected signs of this “cosmic dawn.” By 400 million years after the big bang , the first galaxies were born. In the billions of years since, stars, galaxies, and clusters of galaxies have formed and re-formed—eventually yielding our home galaxy, the Milky Way, and our cosmic home, the solar system.
Even now the universe is expanding , and to astronomers' surprise, the pace of expansion is accelerating. It's thought that this acceleration is driven by a force that repels gravity called dark energy . We still don't know what dark energy is, but it’s thought that it makes up 68 percent of the universe's total matter and energy. Dark matter makes up another 27 percent. In essence, all the matter you've ever seen—from your first love to the stars overhead—makes up less than five percent of the universe.
Related Topics
- BIG BANG THEORY
- SCIENCE AND TECHNOLOGY
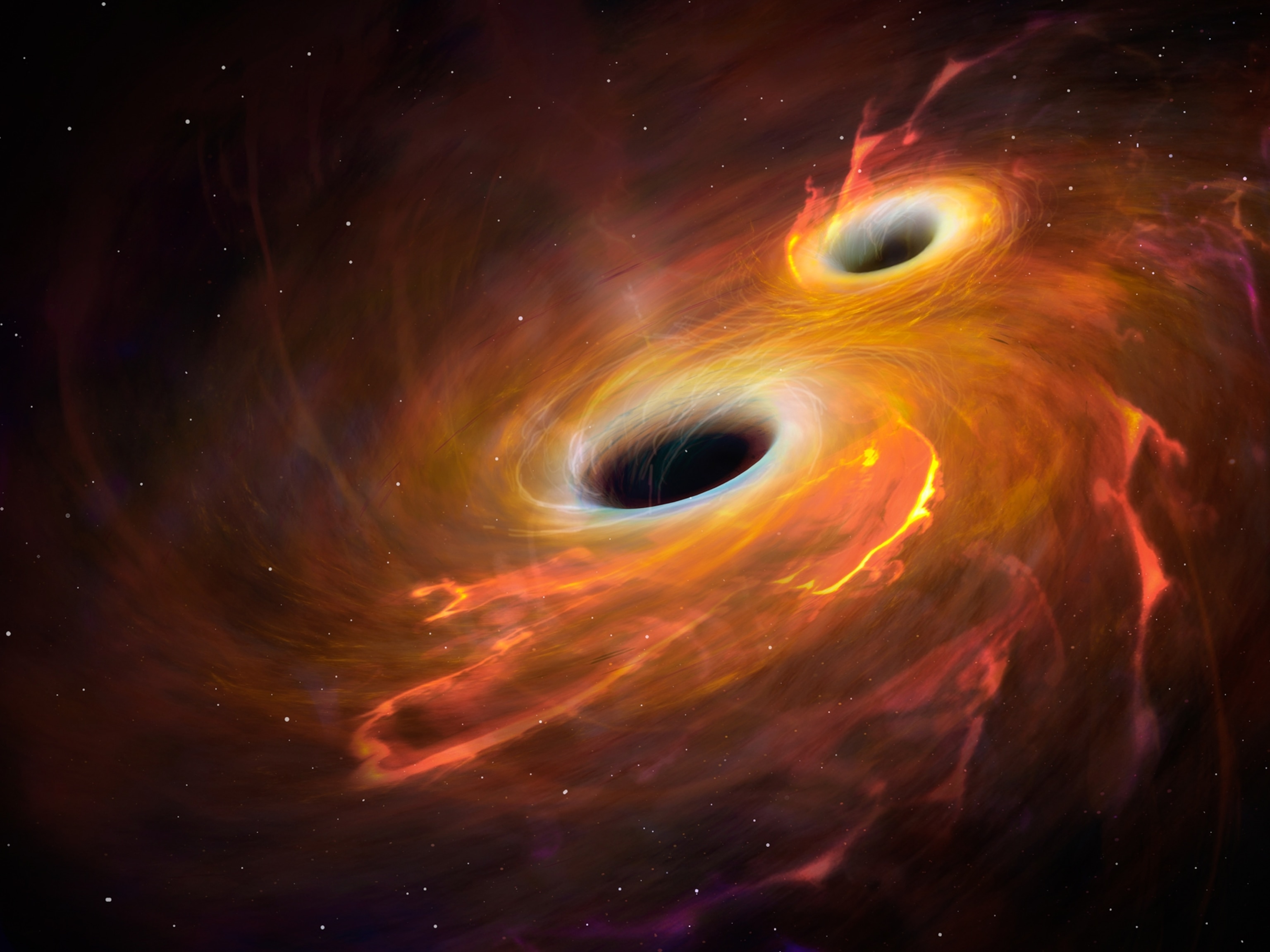
The 11 most astonishing scientific discoveries of 2023
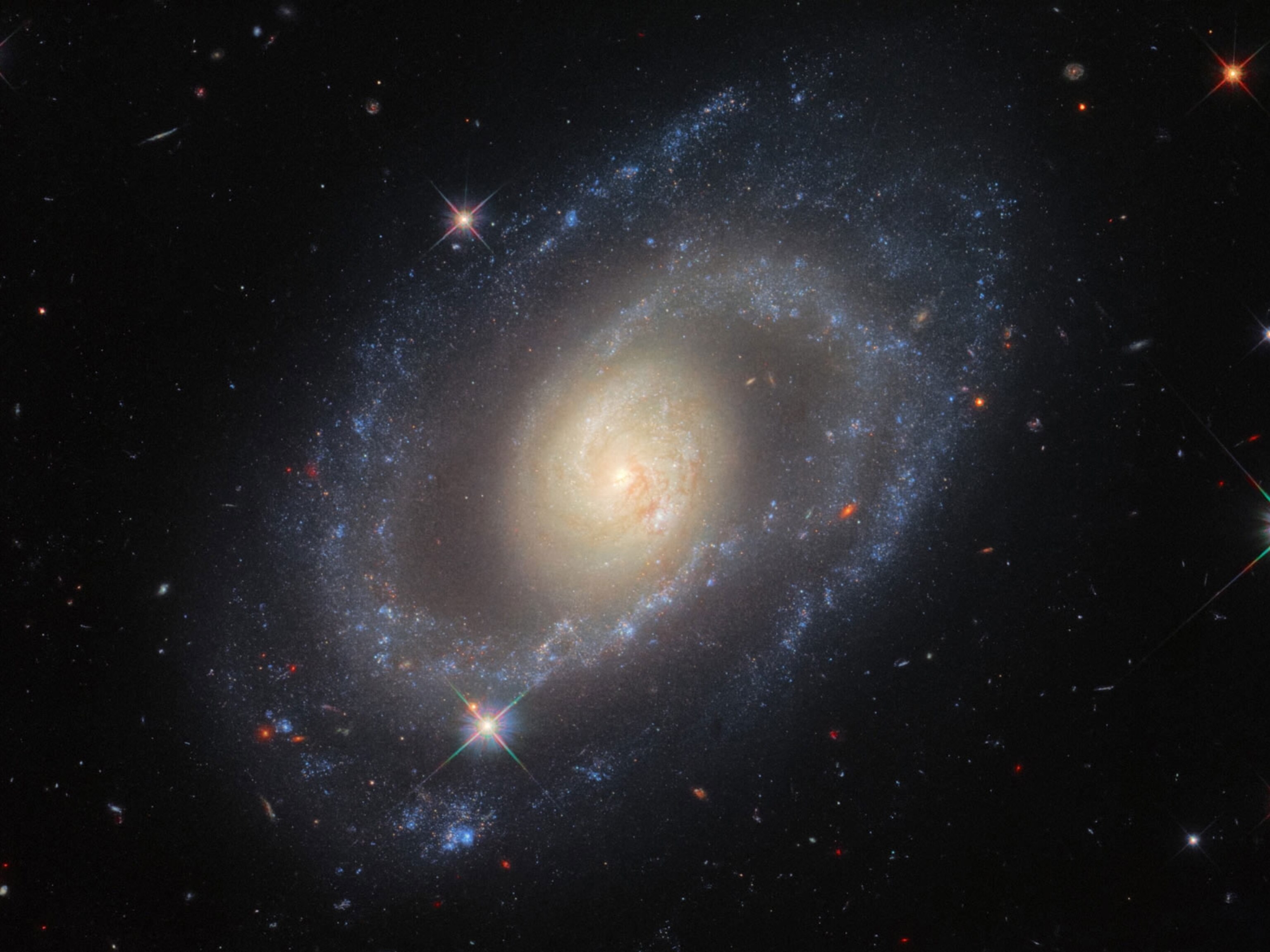
The universe is expanding faster than it should be
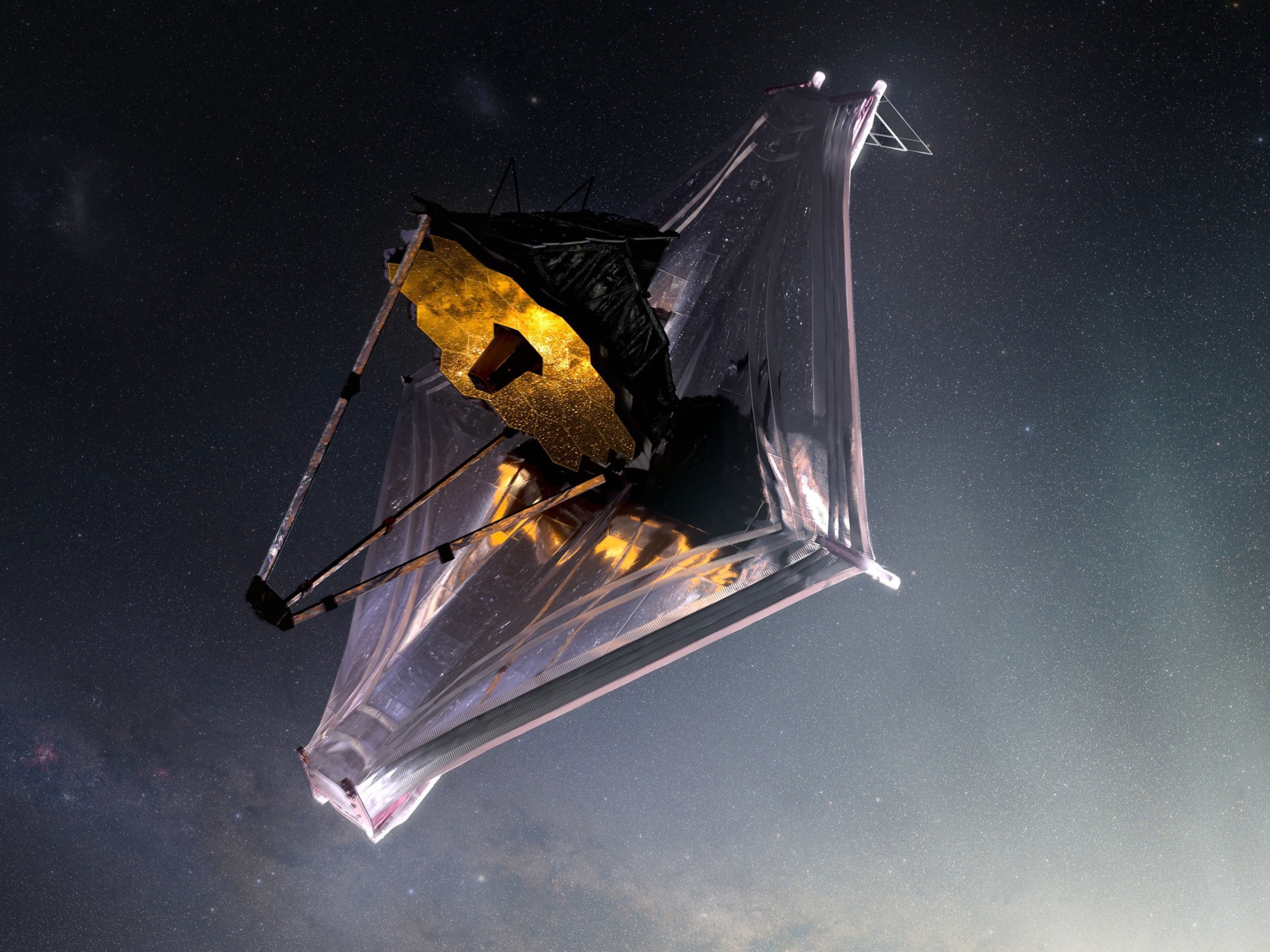
The world's most powerful space telescope has launched at last
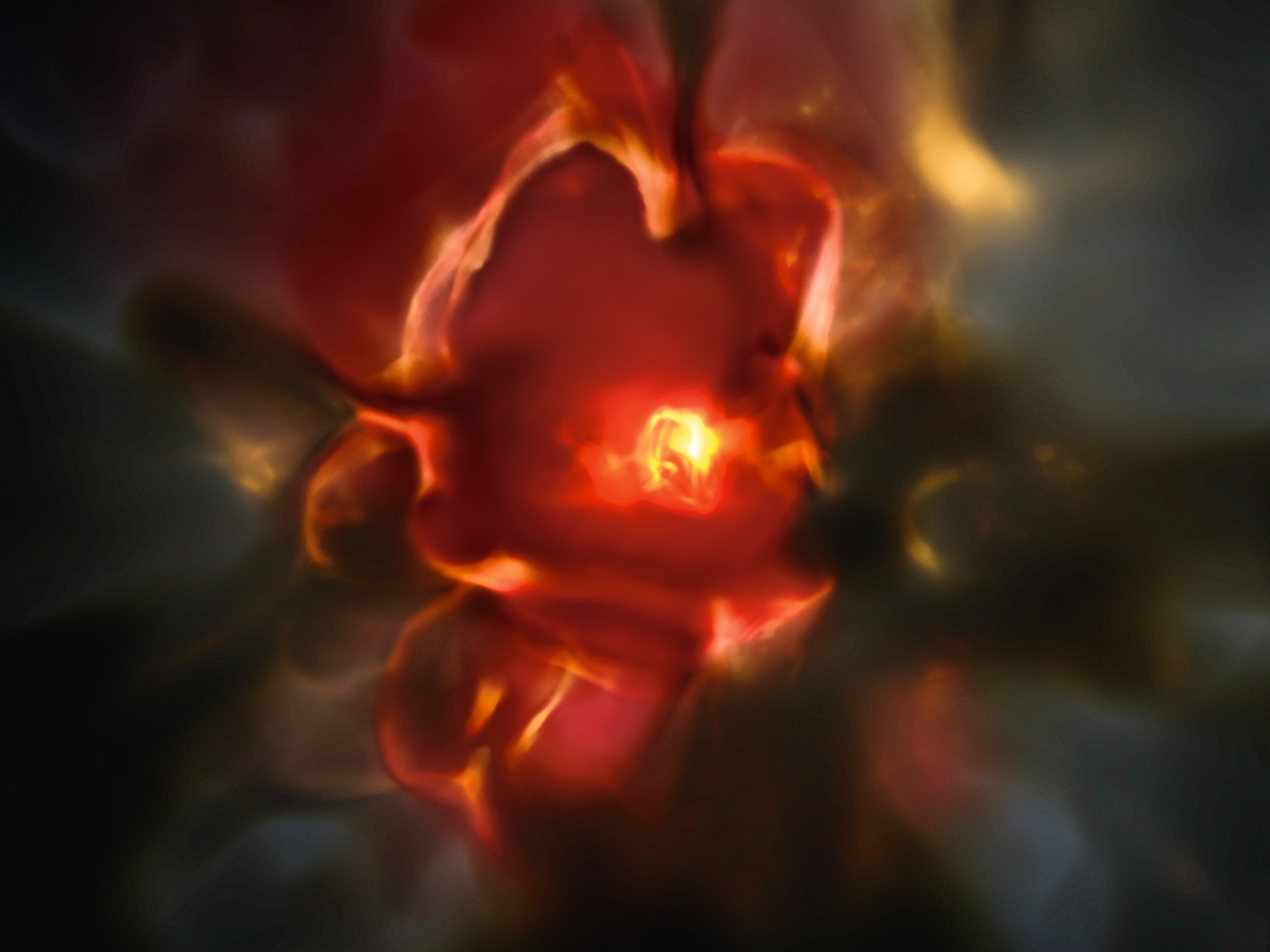
A First Glimpse of the Hidden Cosmos
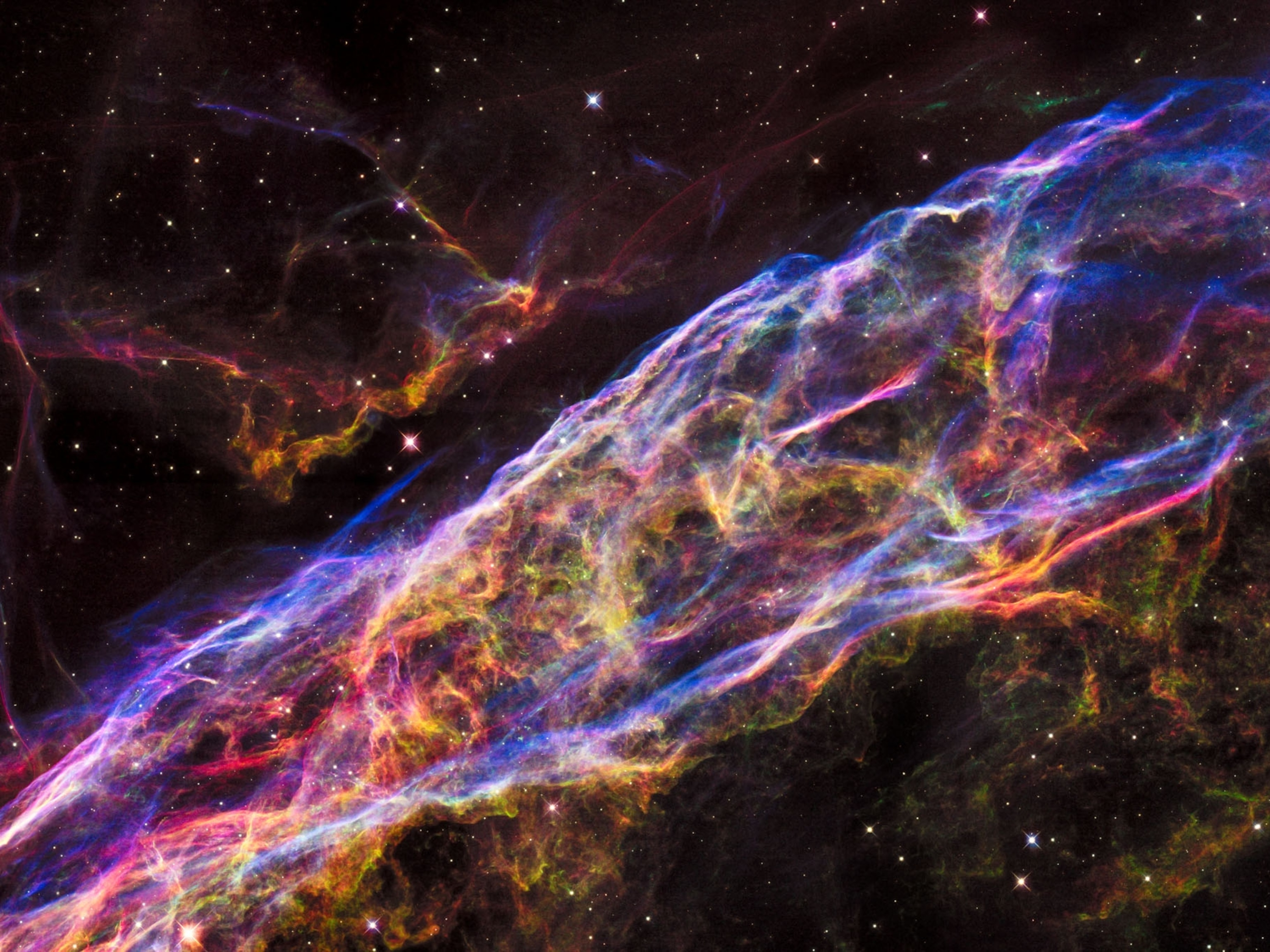
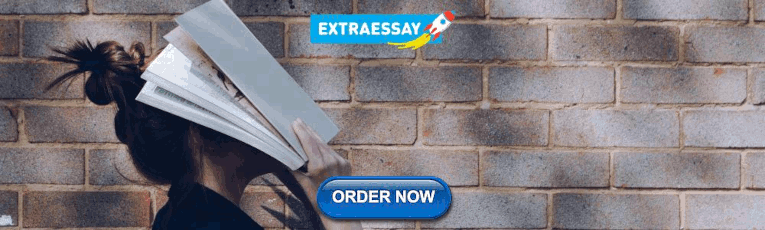
After 30 years, Hubble is still revealing new mysteries of the universe
- Environment
- Perpetual Planet
History & Culture
- History & Culture
- History Magazine
- Mind, Body, Wonder
- Paid Content
- Terms of Use
- Privacy Policy
- Your US State Privacy Rights
- Children's Online Privacy Policy
- Interest-Based Ads
- About Nielsen Measurement
- Do Not Sell or Share My Personal Information
- Nat Geo Home
- Attend a Live Event
- Book a Trip
- Inspire Your Kids
- Shop Nat Geo
- Visit the D.C. Museum
- Learn About Our Impact
- Support Our Mission
- Advertise With Us
- Customer Service
- Renew Subscription
- Manage Your Subscription
- Work at Nat Geo
- Sign Up for Our Newsletters
- Contribute to Protect the Planet
Copyright © 1996-2015 National Geographic Society Copyright © 2015-2024 National Geographic Partners, LLC. All rights reserved
Size of the Universe over time
This graph plots the size of the universe as measured by Hubble telescope observations of exploding stars, called supernovas, at various distances from Earth.
Ann Feild ( STScI )
About the Image
About the object, image formats.

Also see our
- images on esawebb.org

60 Years and Counting
- mysteries of the universe -.
When NASA began 60 years ago, we had questions about the universe humans had been asking since we first looked up into the night sky. In the six decades since, NASA, along with its international partners and thousands of researchers, have expanded our knowledge of the Universe by using a full fleet of telescopes and satellites. From the early probes of the 1950s and 1960s to the great telescopes of the 1990s and 21st century, NASA scientists have been exploring the evolution of the universe from the Big Bang to the present.
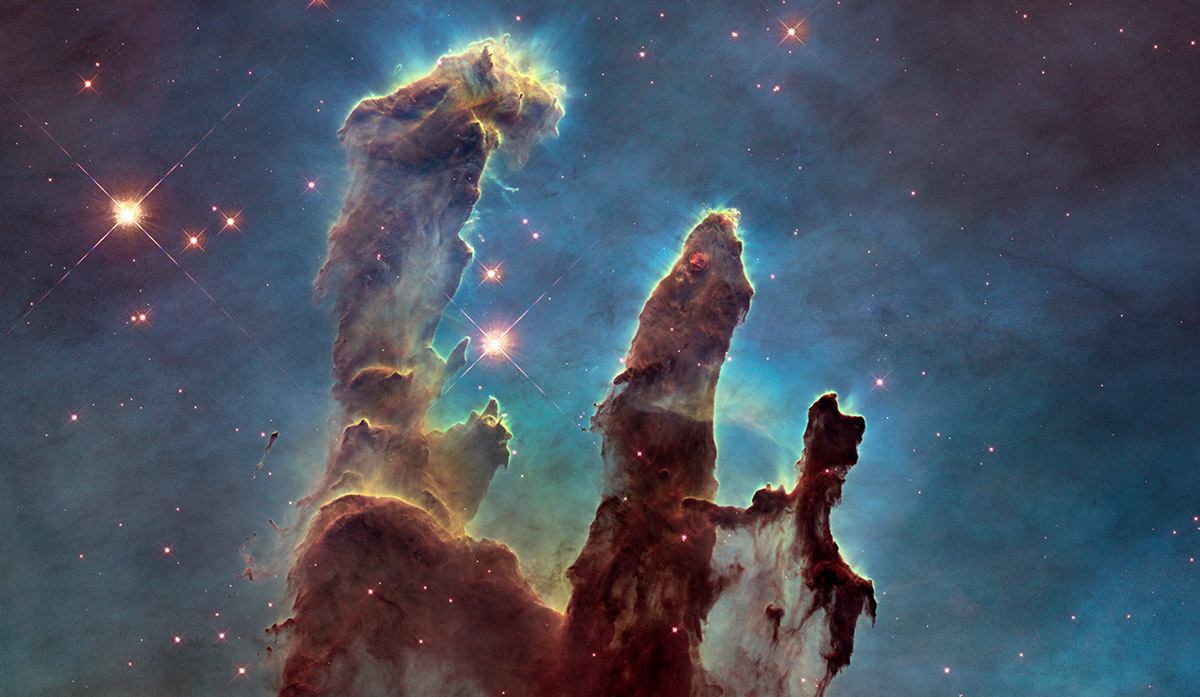
Pillars of Creation, Eagle Nebula, a cloud of gas and dust created by an exploding star from which new stars and planets are forming. Image Credit: NASA/ ESA/The Hubble Heritage Team (STScl/AURA)
The Great Observatories
NASA astronomers use several kinds of telescopes in space and on the ground. Each observes targets like stars, planets, and galaxies, but captures different wavelengths of light using various techniques to add to our understanding of these cosmic phenomenon.
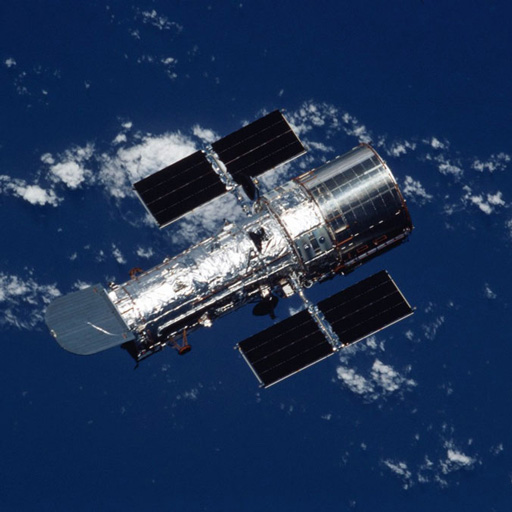
Image Credit: NASA
Since it was launched in 1990, Hubble has forever changed our idea of what the universe looks like. It does not travel to stars, planets or galaxies, but takes pictures of them as it whirls around Earth at about 17,000 mph.
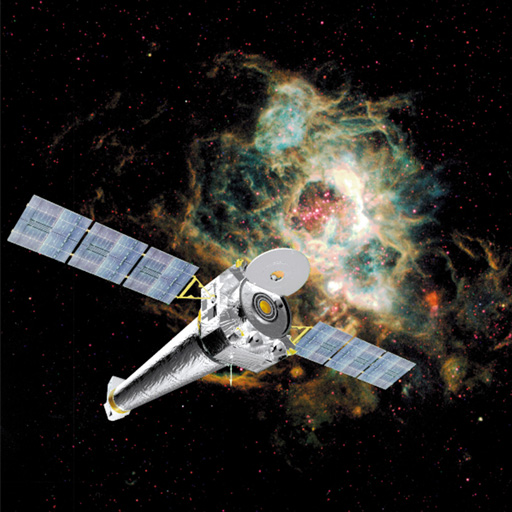
Chandra X-ray Observatory
The Chandra X-ray Observatory allows scientists from around the world to obtain X-ray images of exotic environments to help understand the structure and evolution of the universe. X-rays are produced when matter is heated to millions of degrees. X-ray telescopes can also trace the hot gas from an exploding star or detect X-rays from matter swirling as close as 90 kilometers from the event horizon of a stellar black hole.
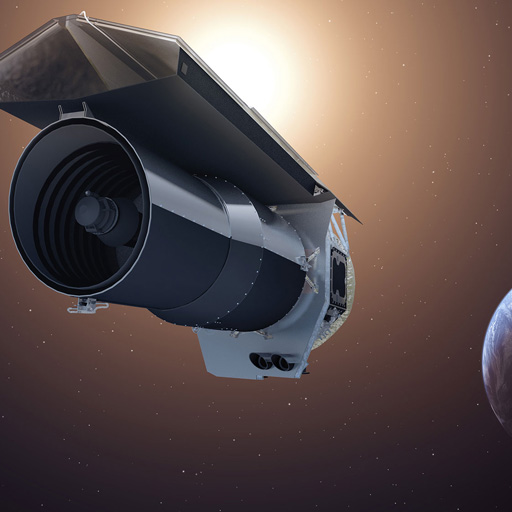
Image Credit: NASA/JPL-Caltech
Spitzer Space Telescope
NASA’s Spitzer Space Telescope, designed to detect primarily heat or infrared radiation, launched in 2003. Spitzer's highly sensitive instruments allow scientists to peer into cosmic regions that are hidden from optical telescopes, including dusty stellar nurseries, the centers of galaxies, and newly forming planetary systems. Spitzer's infrared eyes also allow astronomers to see cooler objects in space, like failed stars (brown dwarfs), exoplanets, giant molecular clouds, and organic molecules that may hold the secret to life on other planets.
Exciting Discoveries
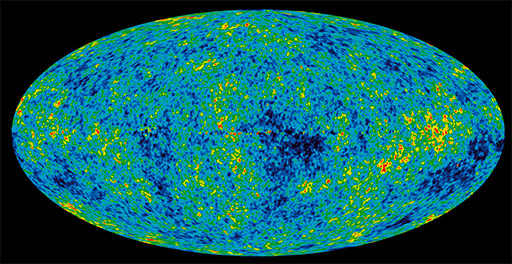
Image of the infant universe 13.7 billion years created from WMAP data, showing differences in temperatures that became “seeds” for galaxies. Image Credit: NASA
The Age of the Universe
The Wilkinson Microwave Anisotropy Probe (WMAP) satellite returned data that allowed astronomers to precisely assess the age of the universe to be 13.77 billion years old and to determine that atoms make up only 4.6 percent of the universe, with the remainder being dark matter and dark energy. Using telescopes like Hubble and Spitzer, scientists also now know how fast the universe is expanding.
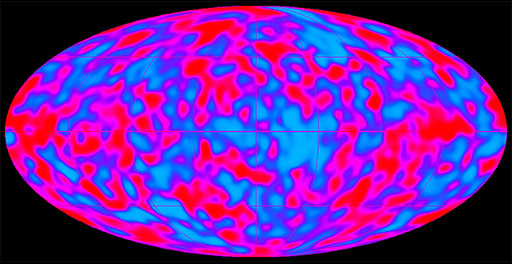
These minute temperature variations (depicted here as varying shades of blue and purple) are linked to slight density variations in the early universe. These variations are believed to have given rise to the structures that populate the universe today: clusters of galaxies, as well as vast, empty regions. Image Credit: NASA
How the Universe Began and Evolved
The Cosmic Background Explorer (COBE), launched in 1989, studied the radiation still left from the Big Bang to better understand how the universe formed. In 2006, John Mather of NASA and George Smoot of the University of California shared the Nobel Prize for Physics for confirming the Big Bang theory using COBE data.
Dark Matter
NASA telescopes have helped us better understand this mysterious, invisible matter that is five times the mass of regular matter. The first direct detection of dark matter was made in 2007 through observations of the Bullet Cluster of galaxies by the Chandra x-ray telescope.
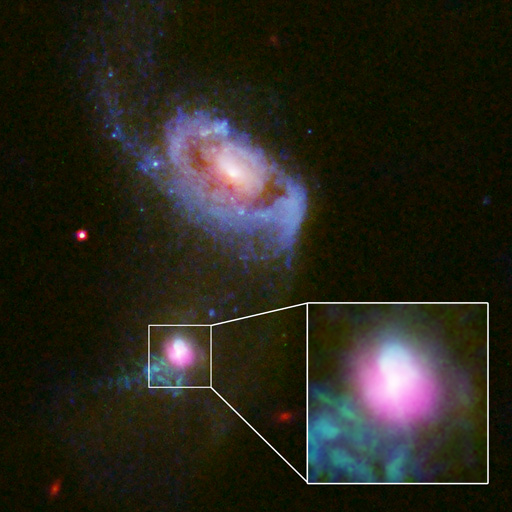
Image Credit: X-ray NASA/CXC/University of Colorado/J. Comerford et al.; Optical: NASA/STScI
Black Holes
Although we can’t “see” black holes, scientists have been able to study them by observing how they interact with the environment around them with telescopes like Swift, Chandra, and Hubble. In 2017, NASA's Swift telescope has mapped the death spiral of a star as it is consumed by a black hole. This year, astronomers using Chandra have discovered evidence for thousands of black holes located near the center of our Milky Way galaxy.
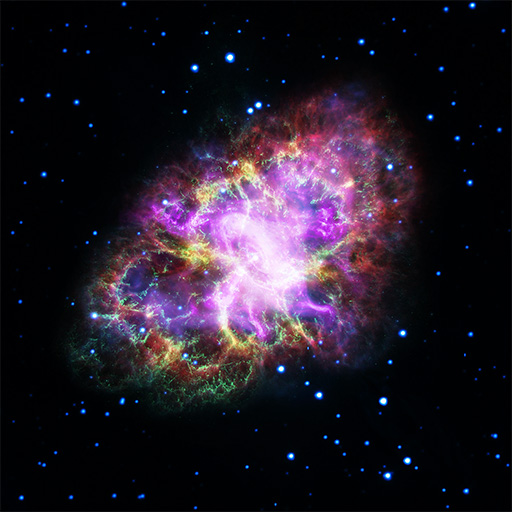
Image Credit: Image Credit: NASA/ESA/G. Dubner (IAFE, CONICET-University of Buenos Aires) et al.; A. Loll et al.; T. Temim et al.; F. Seward et al.; VLA/NRAO/AUI/NSF; Chandra/CXC; Spitzer/JPL-Caltech; XMM-Newton/ESA; and Hubble/STScI
Crab Nebula
Image of the Crab Nebula, combining data from several telescopes. The Crab Nebula, the result of a bright supernova explosion seen by Chinese and other astronomers in the year 1054, is 6,500 light-years from Earth.
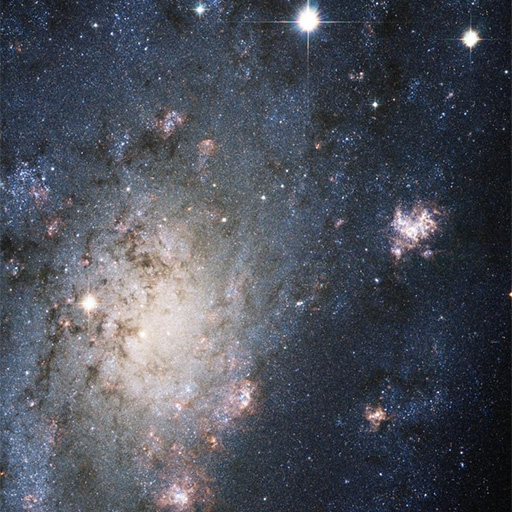
Image Credit: NASA/ESA/A.V. Filippenko (University of California, Berkeley)/P. Challis (Harvard-Smithsonian Center for Astrophysics), et al.
A Bright Supernova
The explosion of a massive star blazes with the light of 200 million Suns in this NASA Hubble Space Telescope image.
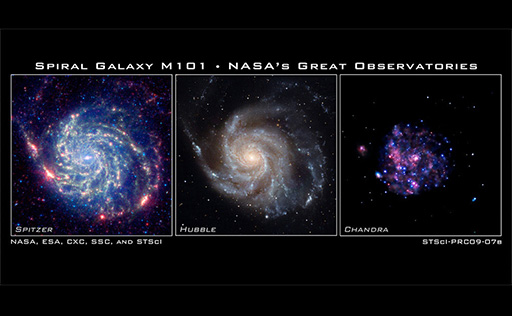
Image Credit: NASA/ESA/CXC/SSC/STScI
Spiral Galaxy M101
Spiral Galaxy M101 viewed from three different NASA telescopes and kinds of light: Spitzer (infrared), Hubble (visible light), and Chandra (X-ray).
A galaxy is a huge collection of gas, dust, and billions of stars and their solar systems, held together by gravity. Some are spiral-shaped like our Milky Way Galaxy; others are smooth and oval shaped. NASA telescopes are helping us learn about how galaxies formed and evolved over time.
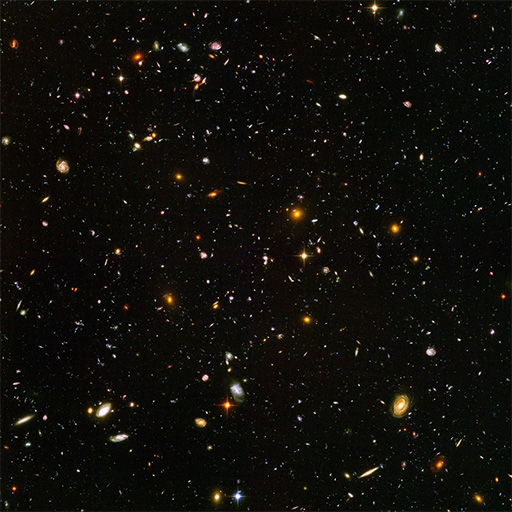
Image Credit: NASA/ESA/S. Beckwith (STScI)/HUDF Team
Thousands of Galaxies
Hubble Space Telescope picture showing thousands of galaxies. Even the tiny dots are entire galaxies.
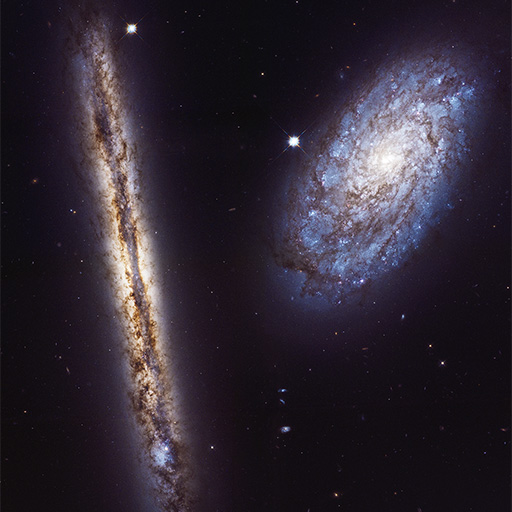
Image Credit: NASA/ESA/M. Mutchler (STScI)
NGC 4302 and NGC 4298
Spiral galaxy pair NGC 4302 and NGC 4298. Astronomers used the Hubble to take a portrait of a stunning pair of spiral galaxies. This starry pair offers a glimpse of what our Milky Way galaxy would look like to an outside observer.
Just 30 years ago, scientists didn’t know if there were planets orbiting other stars besides our own Sun. Now, scientists believe every star likely has at least one exoplanet. They come in a wide variety of sizes, from gas giants larger than Jupiter to small, rocky planets about as big as Earth or Mars. They can be hot enough to boil metal or locked in deep freeze. They can orbit their stars so tightly that a “year” lasts only a few days; they can even orbit two stars at once. Some exoplanets don’t orbit around a star, but wander through the galaxy in permanent darkness. NASA’s Kepler spacecraft and newly-launched Transiting Exoplanet Survey Satellite are helping us find more distant worlds
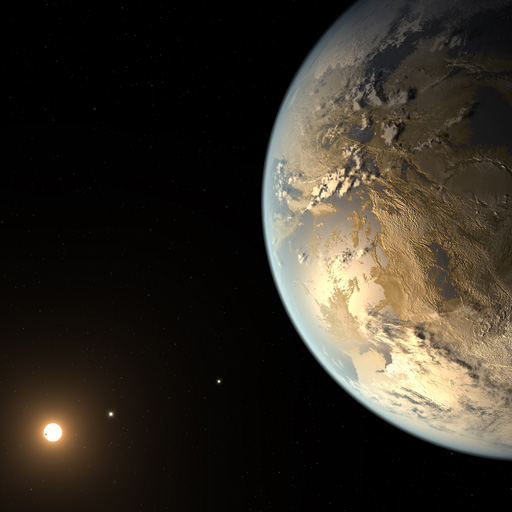
Image Credit: NASA Ames/SETI Institute/JPL-Caltech
Kepler-186f
Kepler-186f, the first rocky exoplanet to be found within the habitable zone—the region around the host star where the temperature is right for liquid water. This planet is also very close in size to Earth. Even though we may not find out what's going on at the surface of this planet anytime soon, it's a strong reminder of why new technologies are being developed that will enable scientists to get a closer look at distant worlds.
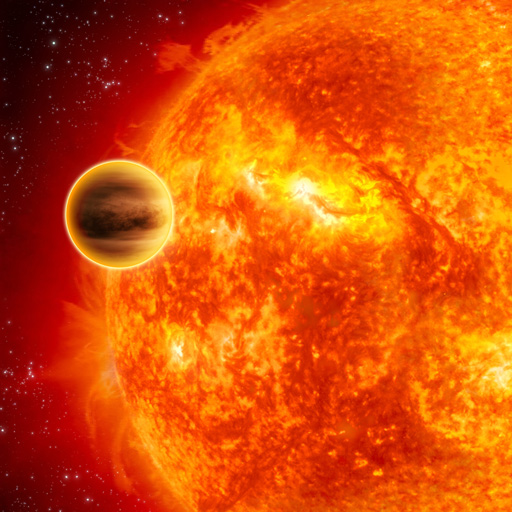
1 Pegasi b. This giant planet, which is about half the mass of Jupiter and orbits its star every four days, was the first confirmed exoplanet around a sun-like star, a discovery that launched a whole new field of exploration.
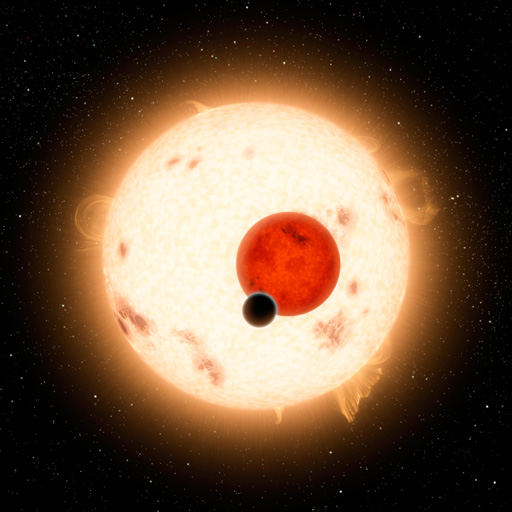
Image Credit: NASA/JPL-Caltech (artist concept)
Kepler-16b. This planet was Kepler's first discovery of a planet that orbits two stars—what is known as a circumbinary planet.
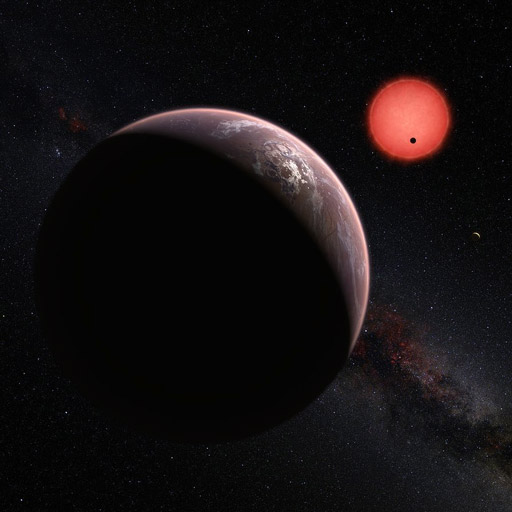
Artist's concept of TRAPPIST-1. Image Credit: NASA
Using Spitzer, scientists found the most number of Earth-sized planets found in the habitable zone of a single star, called TRAPPIST-1. This system of seven rocky worlds–all of them with the potential for water on their surface–is an exciting discovery in the search for life on other worlds. There is the possibility that future study of this unique planetary system could reveal conditions suitable for life.
Origins of the Universe 101
How old is the universe, and how did it begin? Throughout history, countless myths and scientific theories have tried to explain the universe's origins. The most widely accepted explanation is the big bang theory. Learn about the explosion that started it all and how the universe grew from the size of an atom to encompass everything in existence today.
Earth Science, Astronomy
Media Credits
The audio, illustrations, photos, and videos are credited beneath the media asset, except for promotional images, which generally link to another page that contains the media credit. The Rights Holder for media is the person or group credited.
Web Producer
Last updated.
October 19, 2023
User Permissions
For information on user permissions, please read our Terms of Service. If you have questions about how to cite anything on our website in your project or classroom presentation, please contact your teacher. They will best know the preferred format. When you reach out to them, you will need the page title, URL, and the date you accessed the resource.
If a media asset is downloadable, a download button appears in the corner of the media viewer. If no button appears, you cannot download or save the media.
Text on this page is printable and can be used according to our Terms of Service .
Interactives
Any interactives on this page can only be played while you are visiting our website. You cannot download interactives.
Related Resources
- Newsletters
Giant structure in space challenges understanding of the universe

Evrim Yazgin
Evrim Yazgin has a Bachelor of Science majoring in mathematical physics and a Master of Science in physics, both from the University of Melbourne.
About 9.2 billion light-years from Earth is a colossal structure which has confounded astronomers.
The discovery might upend current cosmological theories.
What they’ve found is a 1.3-billion-light-year-across, almost perfect ring of galaxies. No such structure has been seen before. And it doesn’t match any known formation mechanism. It has been dubbed the “Big Ring.”
The discovery was presented at the 243rd meeting of the American Astronomical Society and is detailed in a pre-print paper available on arXiv .
It is the second giant structure found by teams led by Alexia Lopez, an astronomer at the University of Central Lancashire in the UK. The first, a giant arc of galaxies, was unveiled in 2022. That structure is 3.3 billion light-years across and appears in the same region of sky at the same distance from Earth as the Big Ring.
“Neither of these two ultra-large structures is easy to explain in our current understanding of the universe,” Lopez says. “And their ultra-large sizes, distinctive shapes, and cosmological proximity must surely be telling us something important – but what exactly?”
NASA confirms new Saturn moon mission
A possible explanation for the Big Ring, according to Lopez, is “Baryonic Acoustic Oscillations” (BAOs).

“BAOs arise from oscillations in the early universe and today should appear, statistically at least, as spherical shells in the arrangement of galaxies. However, detailed analysis of the Big Ring revealed it is not really compatible with the BAO explanation: the Big Ring is too large and is not spherical.”
Another possibility is the structures are remnants of “defects” in the early universe called cosmic strings.
The structures challenge the so-called “Cosmological Principle.”
“The Cosmological Principle assumes that the part of the universe we can see is viewed as a ‘fair sample’ of what we expect the rest of the universe to be like,” Lopez explains. “We expect matter to be evenly distributed everywhere in space when we view the universe on a large scale, so there should be no noticeable irregularities above a certain size.”
“Cosmologists calculate the current theoretical size limit of structures to be 1.2 billion light-years, yet both of these structures are much larger,” Lopez adds.
“From current cosmological theories we didn’t think structures on this scale were possible. We could expect maybe one exceedingly large structure in all our observable universe. Yet, the Big Ring and the Giant Arc are two huge structures and are even cosmological neighbours, which is extraordinarily fascinating,” Lopez says.
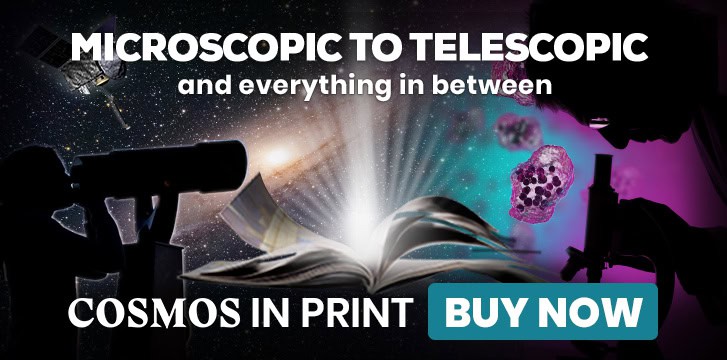
Originally published by Cosmos as Giant structure in space challenges understanding of the universe
Please login to favourite this article.
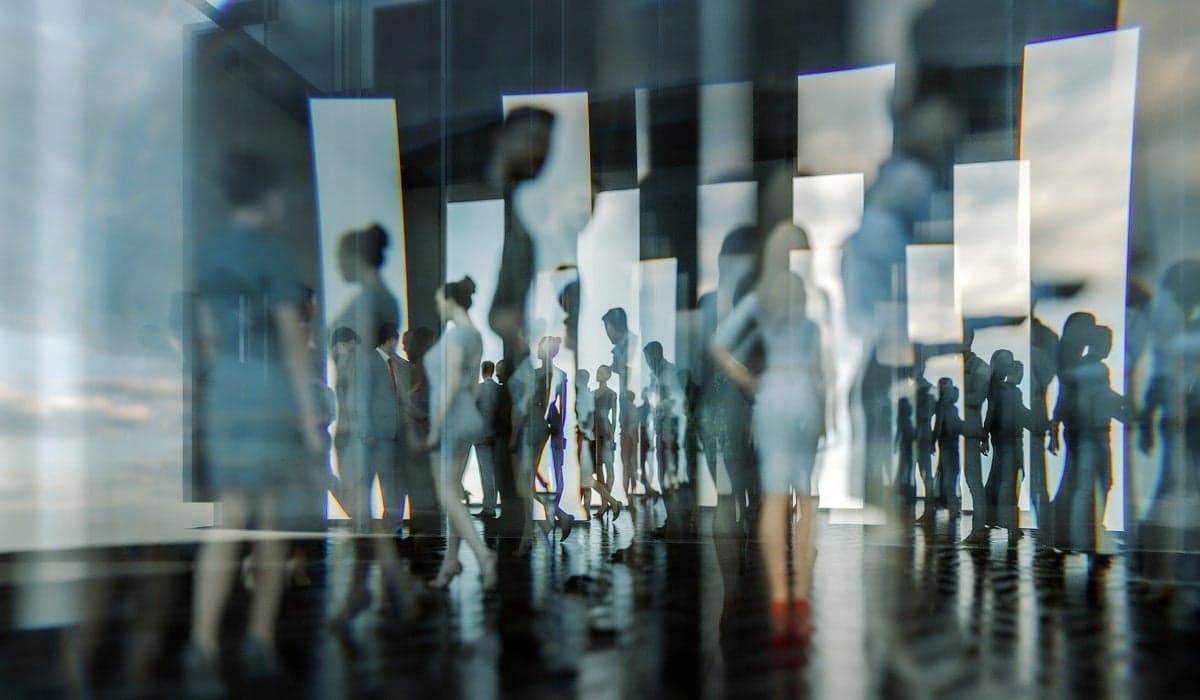
The Universe May Be Shaped Like a Hall of Mirrors, Scientists Say
There's a highway to the edge....
For decades now , scientists have argued about how the universe is shaped, in the sense of complex parameters that govern the rules of space and time. Is it a simple open expanse, like a bigger version of the spaces we're used to? Does it wrap around on itself like a donut? Or something even stranger?
Now, new research published in the journal Physical Review Letters , in the inaugural paper from a new consortium of cosmologists known as the COMPACT Collaboration, found that the "topology" of the universe — the shape of its geometry, basically — is likely anything but simple.
The researchers looked at the universe's cosmic microwave background , which is basically the inherent "glow" of space, dating back to ancient radiation at the dawn of time.
While they didn't nail down any one definite topology for the universe, they did find that data on the universe's background radiation doesn't rule out some seriously exotic shapes — and in fact, we might just live in something akin to an infinite hall of mirrors.
Twist and Shout
Take the main focus of the paper, a shape known as a "3-torus." As the American Physical Society explained in a summary of the paper, that would be like if there was a cube on which each set of opposing sides were connected — meaning that no matter how large the universe appears , if you peer deep enough into its depths, you'll see the back of your head.
The APA described the effect as a "hall of mirrors," adding that "lines of [sight] within a 3-torus form closed loops that return to the viewer, so everything appears to repeat infinitely."
Obviously, this is all just dealing with what's possible, not what actually is — which is why COMPACT is looking to see if they can find a "detectable topological fingerprint" in the cosmic background radiation that might give a more definite answer.
In an interview with the APS, Columbia cosmologist Oliver Philcox, who wasn't involved in the new paper, seemed to agree that any informed guess is worth considering.
"There’s a lot of possible ways the universe could be topologically connected," Philcox said, "and it’s hard to rule them out."
More on cosmological puzzles: Physicists Say They May Have Found a Powerful Glitch in the Universe
Share This Article
Amazon VP Alan Moss Tells Advertisers At Tech Giant's First Upfront That Putting Ads On Prime Video "Connected The Dots Across Our Universe" – Update
UPDATED with additional exec comment. A trio of Amazon ad execs closed the company’s first upfront presentation Tuesday morning in New York, bookending remarks from two senior-level colleagues at the start of the 90-minute show.
Tanner Elton, VP of U.S. Ad Sales, emphasized the company’s difference-making scale, with monthly reach to 200 million monthly viewers of Prime Video. “This is Amazon – we’re the place those customers come to shop and do so much more,” Elton said. “Which means we can do things nobody else can do.”
Sarah Iooss, Director of U.S. Agency Development and Twitch, told ad buyers at Pier 36 that all of them would be able to benefit from the technology and data insights that have long been at the core of the company. “All of you are endemic to Amazon,” she said.
As the pair spoke, screens behind them filled with statistics, including Amazon having a monthly ad-supported reach to 175 million U.S. viewers across Prime Video, Twitch, Fire TV, Freevee and other platforms. Prime Video began running ads earlier this year to all subscribers except those opting to pay a monthly premium to avoid ads.
Elton and Ioss were followed by closing speaker Alan Moss, VP of Global Advertising Sales, Amazon Ads. His run at the company began in 2020 after 13 years at Google as well as exec posts at PayPal and Microsoft. “When I joined Amazon nearly four years ago, the No. 1 question all of you asked me was, ‘When are you going to show ads on Prime Video?’ Well, at Amazon, we like to deliver for all of our customers. By introducing ads on Prime Video, we’ve created the largest ad-supported premium streaming service in the world. We’ve connected the dots across our universe.”
PREVIOUSLY:
Senior Amazon execs Paul Kotas and Mike Hopkins opened the tech giant’s first upfront by detailing the steps toward becoming what Hopkins called a “one-stop shop” for viewers and advertisers.
After Alicia Keys kicked off the presentation at Pier 36 along New York’s East River, Kotas took the stage. The SVP of Amazon Ads began with a quip about his low profile despite having helped build Amazon’s multi-billion-dollar ad business. “Maybe I need to tweak my LinkedIn profile,” he said.
Turning to strategy, he said the company has “created the ability for brands of all types and sizes to reach customers through Prime Video content.” Left unstated but informing the entire splashy affair was Prime Video beginning to run advertising on film and TV titles earlier this year. CEO Andy Jassy, in his annual letter to shareholders last month, said the effort is “off to a great start.” The streaming video offering is a cornerstone of the company’s push into advertising, which has seen revenue climb by more than 20% year-over-year for several consecutive quarters.
“Today is all about how we’re bringing together the vast reach of Prime Video with ad tech that leverages Amazon’s billions of customer signals,” Kotas said. The company’s reach spans the “very top of the funnel to the very bottom for all brands. … We’ve been working toward this moment for years, which is why being on this stage means so much.”
Hopkins, SVP of Prime Video & Amazon Studios, laid out statistics to illustrate Amazon’s size after nearly two decades in the streaming game. With an average of 200 million global customers, 115 million of whom are in the U.S., Prime Video is “the largest global ad-supported streaming service.” Netflix has more overall subscribers, with 270 million, but its ad effort is nascent, while Prime Video’s move into advertising follows a heavy push by corporate siblings like Twitch and Freevee.
“We’ve been working to make Prime Video a one-stop shop for streaming,” Hopkins told ad buyers, “and with your help, we’re making it a one stop advertising destination as well.”
Along with original programming, Hopkins pointed out, Prime Video also features a lengthy roster of third-party streaming outlets, including Max and Crunchyroll, and also has the biggest catalog of film and TV rental and sale titles.
Before moving into the main upfront week alongside media companies, Netflix and YouTube, Amazon staged an event at the NewFronts, with recent editions highlighting a growing presence in live sports. NFL Thursday Night Football is two seasons into an 11-year exclusive deal on Prime Video.
More from Deadline
- 'The Summer I Turned Pretty' Returning To Amazon With Expanded 11-Episode Season 3 In 2025
- 'Tomb Raider' From Phoebe Waller-Bridge Lands Series Order At Amazon
Skip to Content
Venus has almost no water. A new study may reveal why
Illustration of what Venus may have looked like billions of years ago with water, left, and what Venus looks like today, right. (Credits: NASA; NASA/JPL-Caltech)
- Share via Twitter
- Share via Facebook
- Share via LinkedIn
- Share via E-mail
Planetary scientists at CU Boulder have discovered how Venus, Earth’s scalding and uninhabitable neighbor, became so dry.
The new study fills in a big gap in what the researchers call “the water story on Venus.” Using computer simulations, the team found that hydrogen atoms in the planet’s atmosphere go whizzing into space through a process known as “dissociative recombination”—causing Venus to lose roughly twice as much water every day compared to previous estimates.

In Venus' upper atmosphere, hydrogen atoms, orange, whiz into space, leaving behind carbon monoxide molecules, blue and purple. (Credit: Aurore Simonnet/LASP/CU Boulder)
The team published their findings May 6 in the journal Nature. The results could help to explain what happens to water in a host of planets across the galaxy.
“Water is really important for life,” said Eryn Cangi, a research scientist at the Laboratory for Atmospheric and Space Physics (LASP) and co-lead author of the new paper. “We need to understand the conditions that support liquid water in the universe, and that may have produced the very dry state of Venus today.”
Venus, she added, is positively parched. If you took all the water on Earth and spread it over the planet like jam on toast, you’d get a liquid layer roughly 3 kilometers (1.9 miles) deep. If you did the same thing on Venus, where all the water is trapped in the air, you’d wind up with only 3 centimeters (1.2 inches), barely enough to get your toes wet.
“Venus has 100,000 times less water than the Earth, even though it’s basically the same size and mass,” said Michael Chaffin, co-lead author of the study and a research scientist at LASP.
In the current study, the researchers used computer models to understand Venus as a gigantic chemistry laboratory, zooming in on the diverse reactions that occur in the planet’s swirling atmosphere. The group reports that a molecule called HCO+ (an ion made up of one atom each of hydrogen, carbon and oxygen) high in Venus’ atmosphere may be the culprit behind the planet’s escaping water.
For Cangi, co-lead author of the research, the findings reveal new hints about why Venus, which probably once looked almost identical to Earth, is all but unrecognizable today.
“We’re trying to figure out what little changes occurred on each planet to drive them into these vastly different states,” said Cangi, who earned her doctorate in astrophysical and planetary sciences at CU Boulder in 2023.
Spilling the water
Venus, she noted, wasn’t always such a desert.
Scientists suspect that billions of year ago during the formation of Venus, the planet received about as much water as Earth. At some point, catastrophe struck. Clouds of carbon dioxide in Venus’ atmosphere kicked off the most powerful greenhouse effect in the solar system, eventually raising temperatures at the surface to a roasting 900 degrees Fahrenheit. In the process, all of Venus’ water evaporated into steam, and most drifted away into space.
But that ancient evaporation can’t explain why Venus is as dry as it is today, or how it continues to lose water to space.
“As an analogy, say I dumped out the water in my water bottle. There would still be a few droplets left,” Chaffin said.
On Venus, however, almost all of those remaining drops also disappeared. The culprit, according to the new work, is elusive HCO+.
Missions to Venus
Chaffin and Cangi explained that in planetary upper atmospheres, water mixes with carbon dioxide to form this molecule. In previous research, the researchers reported that HCO+ may be responsible for Mars losing a big chunk of its water.

Illustration of NASA's DAVINCI probe falling to the surface of Venus. (Credit: NASA GSFC visualization by CI Labs Michael Lentz and others)
Here’s how it works on Venus: HCO+ is produced constantly in the atmosphere, but individual ions don’t survive for long. Electrons in the atmosphere find these ions, and recombine to split the ions in two. In the process, hydrogen atoms zip away and may even escape into space entirely—robbing Venus of one of the two components of water.
In the new study, the group calculated that the only way to explain Venus’ dry state was if the planet hosted larger than expected volumes of HCO+ in its atmosphere. There is one twist to the team’s findings. Scientists have never observed HCO+ around Venus. Chaffin and Cangi suggest that’s because they’ve never had the instruments to properly look.
While dozens of missions have visited Mars in recent decades, far fewer spacecraft have traveled to the second planet from the sun. None have carried instruments capable of detecting the HCO+ that powers the team’s newly discovered escape route.
“One of the surprising conclusions of this work is that HCO+ should actually be among the most abundant ions in the Venus atmosphere,” Chaffin said.
In recent years, however, a growing number of scientists have set their sights on Venus. NASA’s planned Deep Atmosphere Venus Investigation of Noble gases, Chemistry, and Imaging (DAVINCI) mission, for example, will drop a probe through the planet’s atmosphere all the way to the surface. It’s scheduled to launch by the end of the decade.
DAVINCI won’t be able to detect HCO+, either, but the researchers are hopeful that a future mission might—revealing another key piece of the story of water on Venus.
“There haven’t been many missions to Venus,” Cangi said. “But newly planned missions will leverage decades of collective experience and a flourishing interest in Venus to explore the extremes of planetary atmospheres, evolution and habitability.”
News Headlines
Related articles.
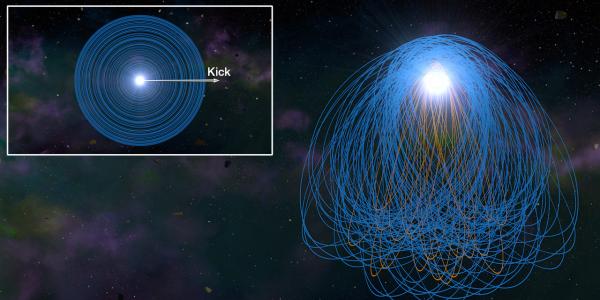
Hungry, hungry white dwarfs: Solving the puzzle of stellar metal pollution
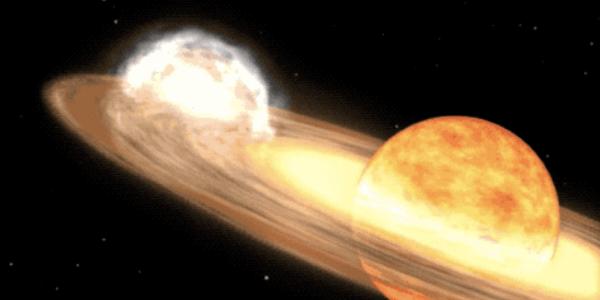
A new star is about to appear in the night sky. Here’s how to catch a glimpse
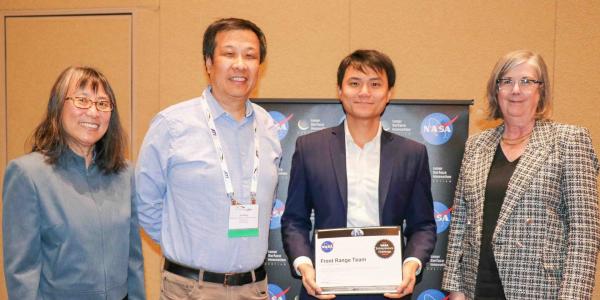
Front Range team wins NASA Entrepreneurs Challenge with innovative idea for lunar service station
- Arts & Humanities
- Business & Entrepreneurship
- Climate & Environment
- Education & Outreach
- Health & Society
- Law & Politics
- Science & Technology
Campus Community
- Administration
- Announcements & Deadlines
- Career Development
- Getting Involved
- Mind & Body
Events & Exhibits
- Arts & Culture
- Conferences
- Lectures & Presentations
- Performances & Concerts
- Sports & Recreation
- Workshops & Seminars
Subscribe to CUBT
Sign up for Alerts
Administrative eMemos
Buff Bulletin Board
Events Calendar
Thank you for visiting nature.com. You are using a browser version with limited support for CSS. To obtain the best experience, we recommend you use a more up to date browser (or turn off compatibility mode in Internet Explorer). In the meantime, to ensure continued support, we are displaying the site without styles and JavaScript.
- View all journals
- Explore content
- About the journal
- Publish with us
- Sign up for alerts
- Published: 14 May 2024
The aged tumor microenvironment limits T cell control of cancer
- Alex C. Y. Chen ORCID: orcid.org/0000-0002-8358-5408 1 , 2 , 3 , 4 ,
- Sneha Jaiswal 1 , 5 na1 ,
- Daniela Martinez 1 , 2 , 3 , 4 na1 ,
- Cansu Yerinde 1 , 2 , 3 , 4 ,
- Keely Ji 1 , 2 , 3 , 4 ,
- Velita Miranda 1 ,
- Megan E. Fung 6 ,
- Sarah A. Weiss ORCID: orcid.org/0000-0002-4155-0346 6 ,
- Maria Zschummel 1 , 2 , 3 , 4 ,
- Kazuhiro Taguchi ORCID: orcid.org/0000-0003-1049-4203 2 , 4 ,
- Christopher S. Garris ORCID: orcid.org/0000-0002-5764-6203 7 , 8 ,
- Thorsten R. Mempel ORCID: orcid.org/0000-0001-8635-5424 2 , 4 ,
- Nir Hacohen 1 , 2 , 3 &
- Debattama R. Sen ORCID: orcid.org/0000-0002-0947-8284 1 , 2 , 3 , 4
Nature Immunology ( 2024 ) Cite this article
Metrics details
- Cytotoxic T cells
- Immunotherapy
- Tumour immunology
The etiology and effect of age-related immune dysfunction in cancer is not completely understood. Here we show that limited priming of CD8 + T cells in the aged tumor microenvironment (TME) outweighs cell-intrinsic defects in limiting tumor control. Increased tumor growth in aging is associated with reduced CD8 + T cell infiltration and function. Transfer of T cells from young mice does not restore tumor control in aged mice owing to rapid induction of T cell dysfunction. Cell-extrinsic signals in the aged TME drive a tumor-infiltrating age-associated dysfunctional (T TAD ) cell state that is functionally, transcriptionally and epigenetically distinct from canonical T cell exhaustion. Altered natural killer cell–dendritic cell–CD8 + T cell cross-talk in aged tumors impairs T cell priming by conventional type 1 dendritic cells and promotes T TAD cell formation. Aged mice are thereby unable to benefit from therapeutic tumor vaccination. Critically, myeloid-targeted therapy to reinvigorate conventional type 1 dendritic cells can improve tumor control and restore CD8 + T cell immunity in aging.
This is a preview of subscription content, access via your institution
Access options
Access Nature and 54 other Nature Portfolio journals
Get Nature+, our best-value online-access subscription
24,99 € / 30 days
cancel any time
Subscribe to this journal
Receive 12 print issues and online access
195,33 € per year
only 16,28 € per issue
Buy this article
- Purchase on Springer Link
- Instant access to full article PDF
Prices may be subject to local taxes which are calculated during checkout
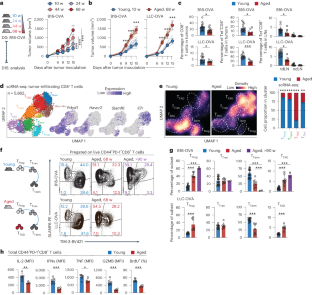
Data availability
Sequence data (scRNA-seq and ATAC-seq) have been deposited in the Gene Expression Omnibus under accession codes GSE255373 and GSE255230 . All human scRNA-seq datasets analyzed in this study have been previously published and are publicly available ( GSE179994 , GSE115978 and E-MTAB-8107 ). The gene sets analyzed with GSEA are available through the Broad Institute Molecular Signatures Database. Source data are provided with this paper.
Code availability
No custom code beyond adaptation of existing software packages were used in this study. All code will be provided upon reasonable request by the corresponding author.
Berben, L., Floris, G., Wildiers, H. & Hatse, S. Cancer and aging: two tightly interconnected biological processes. Cancers 13 , 1400 (2021).
Article CAS PubMed PubMed Central Google Scholar
Tsukamoto, H., Senju, S., Matsumura, K., Swain, S. L. & Nishimura, Y. IL-6-mediated environmental conditioning of defective T H 1 differentiation dampens antitumour immune responses in old age. Nat. Commun. 6 , 6702 (2015).
Article CAS PubMed Google Scholar
Sade-Feldman, M. et al. Defining T cell states associated with response to checkpoint immunotherapy in melanoma. Cell 175 , 998–1013 (2018).
Schreiber, R. D., Old, L. J. & Smyth, M. J. Cancer immunoediting: integrating immunity’s roles in cancer suppression and promotion. Science 331 , 1565–1570 (2011).
Goronzy, J. J., Fang, F., Cavanagh, M. M., Qi, Q. & Weyand, C. M. Naive T cell maintenance and function in human aging. J. Immunol. 194 , 4073–4080 (2015).
Moskowitz, D. M. et al. Epigenomics of human CD8 T cell differentiation and aging. Sci. Immunol. 2 , eaag0192 (2017).
Nikolich-Zugich, J. Ageing and life-long maintenance of T-cell subsets in the face of latent persistent infections. Nat. Rev. Immunol. 8 , 512–522 (2008).
Moskophidis, D., Lechner, F., Pircher, H. & Zinkernagel, R. M. Virus persistence in acutely infected immunocompetent mice by exhaustion of antiviral cytotoxic effector T cells. Nature 362 , 758–761 (1993).
Barber, D. L. et al. Restoring function in exhausted CD8 T cells during chronic viral infection. Nature 439 , 682–687 (2006).
Blackburn, S. D. et al. Coregulation of CD8 + T cell exhaustion by multiple inhibitory receptors during chronic viral infection. Nat. Immunol. 10 , 29–37 (2009).
Miller, B. C. et al. Subsets of exhausted CD8 + T cells differentially mediate tumor control and respond to checkpoint blockade. Nat. Immunol. 20 , 326–336 (2019).
Zheng, L. et al. Pan-cancer single-cell landscape of tumor-infiltrating T cells. Science 374 , abe6474 (2021).
Article PubMed Google Scholar
Goronzy, J. J. & Weyand, C. M. Successful and maladaptive T cell aging. Immunity 46 , 364–378 (2017).
Pereira, B. I. et al. Sestrins induce natural killer function in senescent-like CD8 + T cells. Nat. Immunol. 21 , 684–694 (2020).
Palovics, R. et al. Molecular hallmarks of heterochronic parabiosis at single-cell resolution. Nature 603 , 309–314 (2022).
Akbar, A. N. & Henson, S. M. Are senescence and exhaustion intertwined or unrelated processes that compromise immunity? Nat. Rev. Immunol. 11 , 289–295 (2011).
Mogilenko, D. A. et al. Comprehensive profiling of an aging immune system reveals clonal GZMK + CD8 + T cells as conserved hallmark of inflammaging. Immunity 54 , 99–115 (2021).
Dutta, S. & Sengupta, P. Men and mice: relating their ages. Life Sci. 152 , 244–248 (2016).
White, M. C. et al. Age and cancer risk: a potentially modifiable relationship. Am. J. Prev. Med. 46 , S7–S15 (2014).
Article PubMed PubMed Central Google Scholar
Scott, A. C. et al. TOX is a critical regulator of tumour-specific T cell differentiation. Nature 571 , 270–274 (2019).
Pritykin, Y. et al. A unified atlas of CD8 T cell dysfunctional states in cancer and infection. Mol. Cell 81 , 2477–2493 (2021).
Quinn, K. M. et al. Age-related decline in primary CD8 + T cell responses is associated with the development of senescence in virtual memory CD8 + T cells. Cell Rep. 23 , 3512–3524 (2018).
Zhang, H. et al. Aging-associated HELIOS deficiency in naive CD4 + T cells alters chromatin remodeling and promotes effector cell responses. Nat. Immunol. 24 , 96–109 (2023).
Parish, I. A. et al. The molecular signature of CD8 + T cells undergoing deletional tolerance. Blood 113 , 4575–4585 (2009).
Zhang, H., Weyand, C. M. & Goronzy, J. J. Hallmarks of the aging T-cell system. FEBS J. 288 , 7123–7142 (2021).
Cannarile, M. A. et al. Transcriptional regulator Id2 mediates CD8 + T cell immunity. Nat. Immunol. 7 , 1317–1325 (2006).
Yang, C. Y. et al. The transcriptional regulators Id2 and Id3 control the formation of distinct memory CD8 + T cell subsets. Nat. Immunol. 12 , 1221–1229 (2011).
Gautam, S. et al. The transcription factor c-Myb regulates CD8 + T cell stemness and antitumor immunity. Nat. Immunol. 20 , 337–349 (2019).
Tsui, C. et al. MYB orchestrates T cell exhaustion and response to checkpoint inhibition. Nature 609 , 354–360 (2022).
Wherry, E. J. et al. Molecular signature of CD8 + T cell exhaustion during chronic viral infection. Immunity 27 , 670–684 (2007).
Saul, D. et al. A new gene set identifies senescent cells and predicts senescence-associated pathways across tissues. Nat. Commun. 13 , 4827 (2022).
Yates, K. B. et al. Epigenetic scars of CD8 + T cell exhaustion persist after cure of chronic infection in humans. Nat. Immunol. 22 , 1020–1029 (2021).
Whyte, W. A. et al. Master transcription factors and mediator establish super-enhancers at key cell identity genes. Cell 153 , 307–319 (2013).
Vahedi, G. et al. Super-enhancers delineate disease-associated regulatory nodes in T cells. Nature 520 , 558–562 (2015).
Shin, H. et al. A role for the transcriptional repressor Blimp-1 in CD8 + T cell exhaustion during chronic viral infection. Immunity 31 , 309–320 (2009).
Wu, J. E. et al. In vitro modeling of CD8 + T cell exhaustion enables CRISPR screening to reveal a role for BHLHE40. Sci. Immunol. 8 , eade3369 (2023).
Giordano, M. et al. Molecular profiling of CD8 T cells in autochthonous melanoma identifies Maf as driver of exhaustion. EMBO J. 34 , 2042–2058 (2015).
Moore, M. J. et al. ZFP36 RNA-binding proteins restrain T cell activation and anti-viral immunity. eLife 7 , e33057 (2018).
Petkau, G. et al. The timing of differentiation and potency of CD8 effector function is set by RNA binding proteins. Nat. Commun. 13 , 2274 (2022).
Khan, K. et al. Unravelling structure, localization, and genetic crosstalk of KLF3 in human breast cancer. Biomed. Res. Int. 2020 , 1354381 (2020).
Ilsley, M. D. et al. Kruppel-like factors compete for promoters and enhancers to fine-tune transcription. Nucleic Acids Res. 45 , 6572–6588 (2017).
Shang, W. et al. Genome-wide CRISPR screen identifies FAM49B as a key regulator of actin dynamics and T cell activation. Proc. Natl Acad. Sci. USA 115 , E4051–E4060 (2018).
Liu, B. et al. Temporal single-cell tracing reveals clonal revival and expansion of precursor exhausted T cells during anti-PD-1 therapy in lung cancer. Nat. Cancer 3 , 108–121 (2022).
Qian, J. et al. A pan-cancer blueprint of the heterogeneous tumor microenvironment revealed by single-cell profiling. Cell Res. 30 , 745–762 (2020).
Jerby-Arnon, L. et al. A cancer cell program promotes T cell exclusion and resistance to checkpoint blockade. Cell 175 , 984–997 (2018).
Bonacina, F. et al. Myeloid apolipoprotein E controls dendritic cell antigen presentation and T cell activation. Nat. Commun. 9 , 3083 (2018).
Hildner, K. et al. BATF3 deficiency reveals a critical role for CD8α + dendritic cells in cytotoxic T cell immunity. Science 322 , 1097–1100 (2008).
Schenkel, J. M. et al. Conventional type I dendritic cells maintain a reservoir of proliferative tumor-antigen specific TCF-1 + CD8 + T cells in tumor-draining lymph nodes. Immunity 54 , 2338–2353 (2021).
Bottcher, J. P. et al. NK cells stimulate recruitment of cDC1 into the tumor microenvironment promoting cancer immune control. Cell 172 , 1022–1037 (2018).
Dahling, S. et al. Type 1 conventional dendritic cells maintain and guide the differentiation of precursors of exhausted T cells in distinct cellular niches. Immunity 55 , 656–670 (2022).
Vonderheide, R. H. CD40 agonist antibodies in cancer immunotherapy. Annu. Rev. Med. 71 , 47–58 (2020).
Garris, C. S., Wong, J. L., Ravetch, J. V. & Knorr, D. A. Dendritic cell targeting with Fc-enhanced CD40 antibody agonists induces durable antitumor immunity in humanized mouse models of bladder cancer. Sci. Transl. Med . 13 , eabd1346 (2021).
Sceneay, J. et al. Interferon signaling is diminished with age and is associated with immune checkpoint blockade efficacy in triple-negative breast cancer. Cancer Discov. 9 , 1208–1227 (2019).
Al-Habsi, M. et al. Spermidine activates mitochondrial trifunctional protein and improves antitumor immunity in mice. Science 378 , eabj3510 (2022).
Nakajima, Y., Chamoto, K., Oura, T. & Honjo, T. Critical role of the CD44 low CD62L low CD8 + T cell subset in restoring antitumor immunity in aged mice. Proc. Natl Acad. Sci. USA 118 , e2103730118 (2021).
Padron, A. et al. Age effects of distinct immune checkpoint blockade treatments in a mouse melanoma model. Exp. Gerontol. 105 , 146–154 (2018).
Kaur, A. et al. sFRP2 in the aged microenvironment drives melanoma metastasis and therapy resistance. Nature 532 , 250–254 (2016).
Fane, M. E. et al. Stromal changes in the aged lung induce an emergence from melanoma dormancy. Nature 606 , 396–405 (2022).
Munshi, N. C. et al. Idecabtagene vicleucel in relapsed and refractory multiple myeloma. N. Engl. J. Med. 384 , 705–716 (2021).
Rodriguez-Otero, P. et al. Ide-cel or standard regimens in relapsed and refractory multiple myeloma. N. Engl. J. Med. 388 , 1002–1014 (2023).
Moller, S. H., Hsueh, P. C., Yu, Y. R., Zhang, L. & Ho, P. C. Metabolic programs tailor T cell immunity in viral infection, cancer, and aging. Cell Metab. 34 , 378–395 (2022).
Yi, H. S. et al. T-cell senescence contributes to abnormal glucose homeostasis in humans and mice. Cell Death Dis. 10 , 249 (2019).
Rojas, L. A. et al. Personalized RNA neoantigen vaccines stimulate T cells in pancreatic cancer. Nature 618 , 144–150 (2023).
Nanishi, E., Levy, O. & Ozonoff, A. Waning effectiveness of SARS-CoV-2 mRNA vaccines in older adults: a rapid review. Hum. Vaccin. Immunother. 18 , 2045857 (2022).
Jo, N. et al. Impaired CD4 + T cell response in older adults is associated with reduced immunogenicity and reactogenicity of mRNA COVID-19 vaccination. Nat. Aging 3 , 82–92 (2023).
Kauffman, K. J. et al. Optimization of lipid nanoparticle formulations for mRNA delivery in vivo with fractional factorial and definitive screening designs. Nano Lett. 15 , 7300–7306 (2015).
Das, R. et al. Hybrid LNP prime dendritic cells for nucleotide delivery. Adv. Sci. 10 , e2303576 (2023).
Article Google Scholar
Corces, M. R. et al. Lineage-specific and single-cell chromatin accessibility charts human hematopoiesis and leukemia evolution. Nat. Genet. 48 , 1193–1203 (2016).
Orchard, P., Kyono, Y., Hensley, J., Kitzman, J. O. & Parker, S. C. J. Quantification, dynamic visualization, and validation of bias in ATAC-seq data with ataqv. Cell Syst. 10 , 298–306 (2020).
Download references
Acknowledgements
We thank all members of the Sen laboratory for thoughtful scientific discussions. We thank the Massachusetts General Hospital Flow Cytometry Core and the Massachusetts General Hospital Animal Research Facility for their assistance. We are grateful for helpful advice from R. Das (Massachusetts General Hospital Center for Systems Biology) for some in vivo immunotherapy experiments. D.R.S. was supported by funding from the Melanoma Research Alliance, the V Foundation for Cancer Research and NIH DP2AI176139. A.C.Y.C. was supported by funding from NIH T32CA921643.
Author information
These authors contributed equally: Sneha Jaiswal, Daniela Martinez.
Authors and Affiliations
Krantz Family Center for Cancer Research, Massachusetts General Hospital, Boston, MA, USA
Alex C. Y. Chen, Sneha Jaiswal, Daniela Martinez, Cansu Yerinde, Keely Ji, Velita Miranda, Maria Zschummel, Nir Hacohen & Debattama R. Sen
Department of Medicine, Harvard Medical School, Boston, MA, USA
Alex C. Y. Chen, Daniela Martinez, Cansu Yerinde, Keely Ji, Maria Zschummel, Kazuhiro Taguchi, Thorsten R. Mempel, Nir Hacohen & Debattama R. Sen
Broad Institute of MIT and Harvard, Cambridge, MA, USA
Alex C. Y. Chen, Daniela Martinez, Cansu Yerinde, Keely Ji, Maria Zschummel, Nir Hacohen & Debattama R. Sen
Center for Immunology and Inflammatory Diseases, Massachusetts General Hospital, Boston, MA, USA
Alex C. Y. Chen, Daniela Martinez, Cansu Yerinde, Keely Ji, Maria Zschummel, Kazuhiro Taguchi, Thorsten R. Mempel & Debattama R. Sen
Carnegie Mellon University, Pittsburgh, PA, USA
Sneha Jaiswal
Department of Immunology, Blavatnik Institute, Harvard Medical School, Boston, MA, USA
Megan E. Fung & Sarah A. Weiss
Center for Systems Biology, Massachusetts General Hospital, Boston, MA, USA
Christopher S. Garris
Department of Pathology, Harvard Medical School, Boston, MA, USA
You can also search for this author in PubMed Google Scholar
Contributions
A.C.Y.C. and D.R.S. conceived the study and designed the experiments. A.C.Y.C., S.J., D.M., C.Y., K.J., V.M., M.E.F., S.A.W., M.Z. and K.T. performed experiments and/or data analysis. C.S.G., T.R.M. and N.H. provided critical reagents and advice. A.C.Y.C., S.J., D.M. and D.R.S. wrote the paper. All authors reviewed and edited the paper. All authors are familiar with the Nature Portfolio Inclusion and Ethics guidelines.
Corresponding author
Correspondence to Debattama R. Sen .
Ethics declarations
Competing interests.
The authors declare no competing interests.
Peer review
Peer review information.
Nature Immunology thanks Jorg Goronzy and the other, anonymous, reviewer(s) for their contribution to the peer review of this work. Primary Handling Editor: N. Bernard, in collaboration with the Nature Immunology team.
Additional information
Publisher’s note Springer Nature remains neutral with regard to jurisdictional claims in published maps and institutional affiliations.
Extended data
Extended data fig. 1 phenotyping of cd8 + t cells in young vs. aged tumors..
Related to Fig. 1 . (a) Representative flow cytometry plots (left) and frequencies of splenic CD8 + T cells that were either naive (PD-1 - CD44 − ; right-top), activated (PD-1 + CD44 + ; right-center), or antigen experienced (PD-1 + Tim-3 + ; right-bottom) cells in mice that ranged in 5-85 weeks of age. (b) Representative flow cytometry gating strategy utilized to quantify tumor-infiltrating CD8 + T cells as well as tetramer (Tet + ) specific, and BrdU + CD8 + T cells in young and aged tumor-bearing mice. (c) Representative flow cytometry gating strategy used to measure lymphatic CD8 + T cells as well as tetramer (Tet + ) specific CD8 + T cells in young and aged tumor-bearing mice. (d) ScRNA-seq UMAP projection of 5862 aged tumor-infiltrating CD8 + T cells defined in Fig. 1d . (e) Corresponding violin plots of scRNA-seq that denote RNA read counts (nFeature_RNA; left), and the percentage of mitochondrial genes per cell (% mitochondrial reads; right) for each cluster. (f) Hashtag of scRNA-seq profiles reveals distinct biological replicates, denoted by color, in young (left) and aged (right) tumor-bearing mice. (g) Expression of indicated genes (grey: low, purple: high). (h) Heatmap shows top cluster-defining genes in each CD8 + T cell cluster/subset identified in Fig. 1d . (i) Quantification of subsets within live tetramer (Tet + ) antigen-experienced (CD44 + PD-1 + ) CD8 + T cells, classified based on the Tim-3 and Slamf6 expression in young (blue, n = 8) and aged (red, n = 4) tumor-bearing mice. (j) Geometric mean fluorescence intensity (MFI) of IL-2, IFN-γ, TNF, Gzmb, and BrdU in live antigen-experienced (CD44 + PD-1 + ) tumor-infiltrating CD8 + T cells from young (blue; n = 7) and aged (red; n = 4) tumor-bearing mice, separated by subsets. For panels a, i and j: Summary of at least two independent experiments. Mean ± s.d. For i and j, significance was calculated using a two-sided Student’s t-test. Asterisks used to indicate significance corresponds to the following: N.S. (not significant, P > 0.05), *P ≤ 0.05, **P ≤ 0.01, and ***P ≤ 0.001).
Source data
Extended data fig. 2 transcriptional profiling of t cell-intrinsic defects in aging..
Related to Fig. 2 . (a) Schematic of single and co-transfers OT-1 CD8 + T cells into young tumor bearing recipient mice and the corresponding representative flow cytometry gating strategy utilized to quantify endogenous and transferred OT-1 CD8 + T cells in young tumor bearing recipient mice. (b) Schematic of young and aged splenic OT-1 CD8 + T cell co-transfer into young recipient mice experiments. scRNA-seq UMAP projection of 7731 tumor-infiltrating OT-1 CD8 + T cells from co-transfer of young and aged splenic OT-1 CD8 + T cell into young recipient mice, colored by cluster. Expression of indicated genes (grey: low expression, purple: high expression). (c) Violin plots that denote RNA read counts (nFeature_RNA; top), total number of genes in each cell (nCount_RNA; top second), and the percentage of mitochondrial genes (% mitochondrial reads; top third) and ribosomal genes (% ribosomal reads; bottom) per cell. (d) Heatmap showing top cluster-defining genes in each CD8 + T cell cluster/subset identified in Fig. 2b . (e) Relative proportion of young (OT-1 Y>Y , n = 5) and aged (OT-1 A>Y , n = 5) CD8 + T cells within progenitor-like exhausted (T Prog ), terminally exhausted (T Term ), and dividing (T Divi ) clusters. (f) Experimental design for in vivo transfer assay to study T Prog formation and maintenance at early time points, such as days 7 and 9, between OT-1 Y>Y (blue) and OT-1 A>Y (red) in young B16-OVA tumor-bearing mice on days 7, 9, and 15. Proportion of T Prog , T Tran , and T Term between OT-1 Y>Y and OT-1 A>Y on days 7, 9, and 15 (n = 9-10). (g) UMAP projection of scRNA-seq profiles from 6231 live splenic OT-1 CD8 + T cells from young and aged mice, colored by cluster (C). C1, young naive OT-1 CD8 + T cells (Young Spl T Naiv ); C2, aged naive OT-1 CD8 + T cells (Aged Spl T Naiv ); C3, aged memory-like OT-1 CD8 + T cells (Aged Spl T Mem-like ); C4, aged effector-like OT-1 CD8 + T cells (Aged Spl T Eff-like ); C5, young effector-like OT-1 CD8 + T cells (young Spl T Eff-like ); C6, aged exhausted-like OT-1 CD8 + T cells (Aged Spl T Exh ). Expression of indicated genes (grey: low expression, purple: high expression). i: Tcf7 , ii: Pdcd1 , iii : Ccr7 , iv : Sell , v : Tox . (h) Heatmap showing top cluster-defining genes in each of splenic OT-1 CD8 + T cell cluster/subset identified in Extended Data Fig. 2e , with a specific focus of the top 7 genes in cluster 2 (C2). (i) UMAP projection of scRNA-seq profiles of young splenic OT-1 (Spl OT-1 Y ), aged splenic OT-1 PD-1 - (Spl OT-1 A ), aged splenic OT-1 PD-1 + (Spl OT-1 A PD-1+ ), and wildtype young splenic (WT Y ) CD8 + T cells. Frequencies of each sample within different cell clusters. Mean ± s.d. For e and f, significance was calculated using a two-sided Student’s t-test. Asterisks used to indicate significance corresponds to the following: N.S. (not significant, P > 0.05), *P ≤ 0.05, **P ≤ 0.01, and ***P ≤ 0.001).
Extended Data Fig. 3 Epigenetic profiling of aged T cell-intrinsic defects at baseline.
Related to Fig. 3 . (a) Transcriptional start site (TSS) enrichment of chromatin accessibility profiles from aged and young pre-transfer splenic as well as tumor-infiltrating OT-1 CD8 + T cell subsets. (b) Representative ATAC-seq tracks of aged (yellow) and young (green) splenic naive OT-1 CD8 + T cells at the indicated gene loci. (c) Pathway enrichment in splenic young OT-1 CD8 + T cells (Spl OT-1 Y , green) and splenic aged OT-1 CD8 + T cells (Spl OT-1 A , yellow) chromatin accessible regions (ChARs). The FDR values (hypergeometric test) are presented as -log10(q-value).
Extended Data Fig. 4 Cell-extrinsic effects of aging on differentiation and function of CD8 + T cells.
Related to Fig. 4 , part 1. (a) Experimental design and tumor growth curves for B16-OVA tumors in young (10w; blue; n = 17) and aged (68w; red; n = 16) recipient mice treated with young spleen OT-1 CD8 + T cells (Spl OT-1 Y ). (b) Frequency of transferred OT-1 CD8 + T cells isolated from young (10w, blue; n = 17) and aged (68w; red; n = 16) recipient B16-OVA tumor-bearing mice. (c) B16-OVA tumor volumes at day 15 from the cell-intrinsic model (left, young mice receiving either young or aged splenic OT-1), and cell-extrinsic model (right, young or aged mice received young spleen OT-1). (d) Experimental design for in vitro killing assay and percentage of cytotoxicity of sorted young (blue) and aged (red) OVA tetramer + (Tet + ) intratumoral CD8 + T cell subsets at 1:1 effector to target (E:T; OT-1: B16-OVA) ratio (n = 4-6). (e) Experimental design for in vivo transfer and T cell differentiation. (f) Schematic of young and aged intratumoral Tet + CD8 + T cell subsets transfer into young recipient mice experiments. The corresponding representative flow cytometry gating strategy is utilized to quantify transferred Tet + CD8 + T cell subsets in young tumor-bearing recipient mice. (g) Frequency of transferred OT-1 CD8 + T cells differentiated into different CD8 + T cell subsets (n = 4-5). Mean ± s.d. For b, d, and g, significance was calculated using a two-sided Student’s t-test. Asterisks used to indicate significance corresponds to the following: N.S. (not significant, P > 0.05), *P ≤ 0.05, **P ≤ 0.01, and ***P ≤ 0.001).
Extended Data Fig. 5 Epigenetic and transcriptional profiling of T cell-extrinsic defects in aged tumors.
Related to Fig. 4 , part 2. (a) ScRNA-seq UMAP projection of 4263 tumor-infiltrating OT-1 CD8 + T cells from transfer of young splenic OT-1 CD8 + T cell into young or aged recipient tumor-bearing mice, colored by cluster. Expression of indicated genes (grey: low, purple: high). (b) Heatmap showing top cluster-defining genes in each CD8 + T cell cluster/subset identified in Fig. 4e . (c) Violin plots that denote RNA read counts (nFeature_RNA; top), total number of genes in each cell (nCount_RNA; top second), and the percentage of mitochondrial genes (% mitochondrial reads; bottom) per cell, related to Fig. 4e, f . (d) Volcano plot of differentially expressed genes between transferred young OT-1 (OT-1 Y ) subsets: progenitor-like exhausted (T Prog ; brown) and terminally exhausted (T Term ; blue) as well as the differentially expressed genes between T Term (blue) and tumor-infiltrating age-associated dysfunctional (T TAD ; red) OT-1 CD8 + T cells. (e) Heatmap showing Z-scored TF regulon activity within cell-type clusters identified by Seurat. (f) Principal component analysis (PCA) projection and transcriptional start site (TSS) enrichment of chromatin accessibility profiles from tumor-infiltrating CD8 + T cell subsets in young and aged tumor-bearing mice. (g) Violin plots that denote RNA read counts (nFeature_RNA; top), total number of genes in each cell (nCount_RNA; top second), related to Fig. 4j–l . (h) Heatmap showing top cluster-defining genes in each CD8 + T cell cluster/subset identified in Fig. 4j . (i) Volcano plot of differentially expressed genes between human tumor-infiltrating T cell subsets: T Term vs. T TAD CD8 + T cells.
Extended Data Fig. 6 Phenotyping of immune cells in young vs. aged tumors.
Related to Fig. 5 . (a) Representative flow cytometry gating strategy (top) utilized to quantify intratumoral CD45 + cells in young and aged tumor-bearing mice, including conventional CD4 + T cells, CD8 + T cells, natural killer (NK) cells, and subsets of dendritic cells (DCs), macrophages (Mac), Ly6C + monocytes (Mono), and conventional dendritic cells type1 (cDC1s) and 2 (cDC2s) within DCs. Representative flow cytometry gating strategy (bottom) utilized to lymphatic CD8 + T cells, tetramer (Tet + ) specific CD8 + T cells, subsets of dendritic cells (DCs), and cDC1/cDC2 within DCs in young and aged tumor-bearing mice. (b) Heatmap shows top cluster-defining genes in each cell cluster/subset identified in Fig. 5c . (c) Volcano plot of differentially expressed genes between intratumoral T, NK, and myeloid cells from young (blue) and aged (red) tumor-bearing mice. (d) All the significant ligand-receptor interactions between NK, cDC1, and CD8 + T cells in young (blue) and aged (red) tumor-bearing mice. The dot color and size represent the calculated communication probability and p-values. (e) Percentage of intratumoral CD8 + T cells and OVA tetramer + (Tet + ) intratumoral CD8 + T cells from B16-OVA tumor-bearing mice with different ages, from 10w, 24w, 44w, up to 68w old (n = 6-8). (f) Percentage of intratumoral CD8 + T cell subsets (such as T Term and T TAD ) from B16-OVA tumor-bearing mice with different ages, from 10w, 24w, 44w, up to 68w old (n = 6-8). Mean ± s.d. For b, d, e, f, and g, significance was calculated using a two-sided Student’s t-test. Asterisks used to indicate significance corresponds to the following: N.S. (not significant, P > 0.05), *P ≤ 0.05, **P ≤ 0.01, and ***P ≤ 0.001).
Extended Data Fig. 7 Transcriptional profiling of CD8 + T cells after myeloid-targeted immunotherapy.
Related to Fig. 6 . (a) ScRNA-seq UMAP projection of 3454 tumor-infiltrating CD8 + T cells defined in Fig. 6h . (b) Expression of indicated genes (grey: low, purple: high). (c) Corresponding violin plots of scRNA-seq that denote RNA read counts (nFeature_RNA; up), and the percentage of mitochondrial genes per cell (% mitochondrial reads; down) for each cluster. (d) Heatmap shows top cluster-defining genes in each CD8 + T cell cluster/subset identified in Fig. 6h . (e) ScRNA-seq UMAP projection of different tumor-infiltrating CD8 + T cell cluster proportion from untreated young/aged, and young/aged treated with CD40 agonist antibody mice. (f) Quantification of naive (T Naiv ), transitory exhausted (T Tran ), IFN-reponse (T IFN ), dividing (T Divi ), and Lars2 + (T Lars2+ ) subsets based on scRNA with individual mouse hashtags (n = 5). (g) Volcano plot of differentially expressed genes between transferred terminally exhausted (T Term ; brown) and different subsets, including tumor-infiltrating age-associated dysfunctional (T TAD ; red), progenitor-like exhausted (T Prog ; golden yellow), memory-like (T Mem-like ), and effector-like (T Eff-like ) subsets. (h) Dot plot of different expression levels of critical surface markers and transcriptional regulators among each CD8 + T cell cluster. Mean ± s.d. For f, significance was calculated using a two-sided Student’s t-test. Asterisks used to indicate significance corresponds to the following: N.S. (not significant, P > 0.05), *P ≤ 0.05, **P ≤ 0.01, and ***P ≤ 0.001).
Supplementary information
Supplementary information.
Supplementary Tables 1–20.
Reporting Summary
Supplementary tables 1–20.
Supplementary Table 1. Gene expression by cluster in scRNA-seq data from Fig. 1d . Each row is a gene; columns include P value, log 2 fold change, percentage of cells with non-zero transcript, Bonferroni correction of observed differences and cluster identity. Supplementary Table 2. Gene expression by cluster in scRNA-seq data from Fig. 2b . Each row is a gene; columns include P value, log 2 fold change, percentage of cells with non-zero transcript, Bonferroni correction of observed differences and cluster identity. Supplementary Table 3. Differential gene expression between aged and young OT-1 T Term cells in scRNA-seq data from Fig. 2d . Each row is a gene; columns include P value, log 2 fold change, percentage of cells with non-zero transcript, Benjamini–Hochberg and FDR correction of observed differences. Supplementary Table 4. Gene expression by cluster in scRNA-seq data from Fig. 2h . Each row is a gene; columns include P value, log 2 fold change, percentage of cells with non-zero transcript, Bonferroni correction of observed differences and cluster identity. Supplementary Table 5. Differential gene expression between aged and young splenic T Naiv cells in scRNA-seq data from Fig. 2i . Each row is a gene; columns include P value, log 2 fold change, percentage of cells with non-zero transcript, Benjamini–Hochberg and FDR correction of observed differences. Supplementary Table 6. GSEA of differential open chromatin regions between aged and young OT-1 T Term cells from Fig. 3c . Each row is a gene set; columns include FDR q value, hypergeometric fold enrichment, –log 10 ( q value) and direction of significant enrichment. Supplementary Table 7. GSEA of differential open chromatin regions between aged and young splenic T Naiv cells from Extended Data Fig. 3c . Each row is a gene set; columns include FDR q value, hypergeometric fold enrichment, –log 10 ( q value) and direction of significant enrichment. Supplementary Table 8. Gene expression by cluster in scRNA-seq data from Fig. 4e . Each row is a gene; columns include P value, log 2 fold change, percentage of cells with non-zero transcript, Bonferroni correction of observed differences and cluster identity. Supplementary Table 9. Differential gene expression between denoted clusters in scRNA-seq data from Extended Data Fig. 5d . Each row is a gene; columns include P value, log 2 fold change, percentage of cells with non-zero transcript, Benjamini–Hochberg and FDR correction of observed differences. Supplementary Table 10. Summary of genes assigned to differential open chromatin regions between endogenous T Term and T TAD cells from aged mice in Fig. 4i . Supplementary Table 11. Summary of publicly available samples used for the human pan-cancer scRNA-seq analysis from Fig. 4j–l . Each row is an individual; columns include dataset, cancer type, participant ID, sex, age, age group, stage, TNM classification, pathological subtype, molecular status, sample type, scRNA-seq ID and treatment. Supplementary Table 12. Gene expression by cluster in scRNA-seq data from Fig. 4j . Each row is a gene; columns include P value, log 2 fold change, percentage of cells with non-zero transcript, Bonferroni correction of observed differences and cluster identity. Supplementary Table 13. Differential gene expression between human T TAD and T Term cells in scRNA-seq data from Extended Data Fig. 5i . Each row is a gene; columns include P value, log 2 fold change, percentage of cells with non-zero transcript, Benjamini–Hochberg and FDR correction of observed differences. Supplementary Table 14. Gene expression by cluster in scRNA-seq data from Extended Data Fig. 6b . Each row is a gene; columns include P value, log 2 fold change, percentage of cells with non-zero transcript, Bonferroni correction of observed differences and cluster identity. Supplementary Table 15. Differential gene expression between aged and young T cells, NK cells and myeloid cells in scRNA-seq data from Extended Data Fig. 6c . Each row is a gene; columns include P value, log 2 fold change, percentage of cells with non-zero transcript, Benjamini–Hochberg and FDR correction of observed differences. Supplementary Table 16. Differential gene expression between aged and young DCs in scRNA-seq data from Fig. 5d . Each row is a gene; columns include P value, log 2 fold change, percentage of cells with non-zero transcript, Benjamini–Hochberg and FDR correction of observed differences. Supplementary Table 17. Differentially enriched interactions between aged and young DCs, NK cells and CD8 + T cells in scRNA-seq data from Extended Data Fig. 6d . Each row is a receptor–ligand interaction; columns include sender–receiver pairing, interaction type, enrichment in young and aged and delta of enrichment. Supplementary Table 18. Gene expression by cluster in scRNA-seq data from Fig. 6h . Each row is a gene; columns include P value, log 2 fold change, percentage of cells with non-zero transcript, Bonferroni correction of observed differences and cluster identity. Supplementary Table 19. Differential gene expression between denoted clusters in scRNA-seq data from Extended Data Fig. 7g . Each row is a gene; columns include P value, log 2 fold change, percentage of cells with non-zero transcript, Benjamini–Hochberg and FDR correction of observed differences. Supplementary Table 20. Flow cytometry antibodies. All antibodies used in this study, listing target protein, conjugated fluorophore, antibody clone, manufacturer and catalog number.
Source Data Figs. 1–6 and Extended Data Figs. 1, 2, 4, 6 and 7
Statistical source data.
Rights and permissions
Springer Nature or its licensor (e.g. a society or other partner) holds exclusive rights to this article under a publishing agreement with the author(s) or other rightsholder(s); author self-archiving of the accepted manuscript version of this article is solely governed by the terms of such publishing agreement and applicable law.
Reprints and permissions
About this article
Cite this article.
Chen, A.C.Y., Jaiswal, S., Martinez, D. et al. The aged tumor microenvironment limits T cell control of cancer. Nat Immunol (2024). https://doi.org/10.1038/s41590-024-01828-7
Download citation
Received : 11 October 2023
Accepted : 27 March 2024
Published : 14 May 2024
DOI : https://doi.org/10.1038/s41590-024-01828-7
Share this article
Anyone you share the following link with will be able to read this content:
Sorry, a shareable link is not currently available for this article.
Provided by the Springer Nature SharedIt content-sharing initiative
Quick links
- Explore articles by subject
- Guide to authors
- Editorial policies
Sign up for the Nature Briefing: Cancer newsletter — what matters in cancer research, free to your inbox weekly.

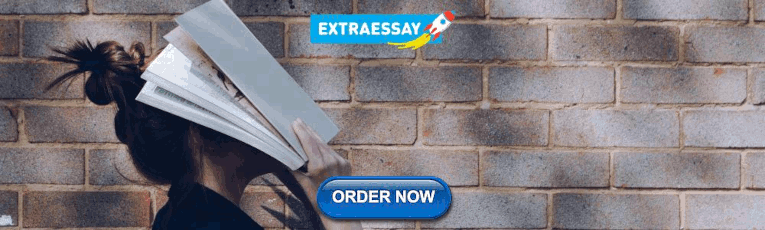
IMAGES
VIDEO
COMMENTS
Scale of Universe is an interactive experience to inspire people to learn about the vast ranges of the visible and invisible world. About us Resources Create with Us! Wiki Objects Community. Hello! Enter your email to subscribe to our newsletter! We have some big things coming and you don't want to miss out. 🤯🚀
The Universe is so big because it is constantly expanding, and it does so at a speed that even exceeds the speed of light. Space itself is actually growing, and this is going on for around 14 billion years or so. In this amount of time, with speed greater than the speed of light, the Universe gradually grew, and it still expands even to this day.
If the universe expanded at the speed of light during inflation, it should be 10^23, or 100 sextillion. One explanation for this, outlined by NASA in 2019, is that dark energy events may have ...
The Universe's History The origin, evolution, and nature of the universe have fascinated and confounded humankind for centuries. New ideas and major discoveries made during the 20th century transformed cosmology - the term for the way we conceptualize and study the universe - although much remains unknown. Here is the history of the universe according […]
For 4K space, and more great History and Science than you'll ever watch, check out our sister network... https://www.magellantv.com/featuredThe universe has ...
The size of the Universe - II: The size of the Universe - III: Scale model of the Universe: The Speed of Light: The Age of the Universe - I: The Age of the Universe - II: The Age of the Universe - III: The Form of the Universe: Hubble's Law and Redshift: Our Three-Dimensional Universe: Does the Universe have an Edge? Space is Curved ...
The universe is all of space and time and their contents. It comprises all of existence, any fundamental interaction, physical process and physical constant, and therefore all forms of energy and matter, and the structures they form, from sub-atomic particles to entire galaxies.Space and time, according to the prevailing cosmological theory of the Big Bang, emerged together 13.787 ± 0.020 ...
The Universe: Size, Shape, and Fate Tom Murphy 9th January 2006 1 The Scale of the Universe Our universe extends staggeringly far beyond our own earthly environment. Trying to grasp the size in any meaningful way is bound to make your brain hurt. We can make analogies to at least understand a few of
The featured interactive flash animation, a modern version of the classic video Powers of Ten , is a new window to many of the known scales of our universe. By moving the scroll bar across the bottom, you can explore a diversity of sizes, while clicking on different items will bring up descriptive information. Tomorrow's picture ...
The Size of the Universe and Where Will We Go? ... The presentations and materials posted to the National Aeronautics and Space Administration Engineering and Safety Center Academy online training are provided for informational purposes only. The use of trademarks, names of manufacturers, or particular items shown or mentioned in the ...
History, Space Science, Scientists and Inventors, Universe. Type. Websites. This website shows how generations of explorers have taken us, step by step, ever farther into the vast expanse of the universe. It is a journey of discovery that has only just begun. Go to Website.
The universe is nearly 14 billion years old, our solar system is 4.6 billion years old, life on Earth has existed for maybe 3.8 billion years, and humans have been around for only a few hundred thousand years. In other words, the universe has existed roughly 56,000 times longer than our species has.
ÐÏ à¡± á> þÿ K þÿÿÿ à á û ü ý þ ÿ ÷ ø À B É Ê Ë Ì Á  À m n o p q r ; ' -.|««u ®=C§
The Universe has not always been the same size. Scientists believe it began in a Big Bang, which took place nearly 14 billion years ago. Since then, the Universe has been expanding outward at very high speed. So the area of space we now see is billions of times bigger than it was when the Universe was very young. The galaxies are also moving ...
Manage Classes & Assignments. Sync with Google Classroom. Create Lessons. Customized Dashboard. Find lessons on Large-scale Structure and Composition of Universe for all grades. Free interactive resources and activities for the classroom and home.
The Size of Space. Made by Neal Agarwal. Swipe left to start Use the Right Arrow Key or Swipe Left to Start. The Size Of Space. Somewhere, something incredible is waiting to be known. - Carl Sagan. Made with by Neal Agarwal. Explore more posts on Neal.fun. Buy me a coffee!
Presentation Transcript. Size and Scale of the Universe Image courtesy of The Cosmic Perspective by Bennett, Donahue, Schneider, & Voit; Addison Wesley, 2002. Earth • Planet where we all live • Comprised primarily of rock • Spherical in shape • 12,700 km in diameter • It would take 17 days to circumnavigate the globe driving a car at ...
Free Google Slides theme, PowerPoint template, and Canva presentation template. If you are an astronomy teacher you have to see this template. It is specially designed to create a fun presentation about this science. It has a clear background on which we have included a multitude of doodle-style illustrations that your audience will love.
Here's the theory: In the first 10^-43 seconds of its existence, the universe was very compact, less than a million billion billionth the size of a single atom.
Age and size of the Universe The lives of stars The solar neighbourhood Exoplanets and proto-planetary discs Black Holes, Quasars, and Active Galaxies Formation of stars Composition of the Universe Gravitational lenses Multi-messenger astronomy Europe & Hubble History Timeline Launch 1990
From the early probes of the 1950s and 1960s to the great telescopes of the 1990s and 21st century, NASA scientists have been exploring the evolution of the universe from the Big Bang to the present. NASA 60th: What's Out There. Watch on. Pillars of Creation, Eagle Nebula, a cloud of gas and dust created by an exploding star from which new ...
Origins of the Universe 101. How old is the universe, and how did it begin? Throughout history, countless myths and scientific theories have tried to explain the universe's origins. The most widely accepted explanation is the big bang theory. Learn about the explosion that started it all and how the universe grew from the size of an atom to ...
Download the Universe Information PowerPoint and read through the facts on each slide with your child. This PowerPoint explains the big bang theory in a child-friendly way and contains information about how the Universe was formed and what it's made of. Encourage your child to ask questions and make comments on what they're learning. It is also a great resource for introducing them to ...
About 9.2 billion light-years from Earth is a colossal structure in the universe which has confounded astronomers. ... "Cosmologists calculate the current theoretical size limit of structures to ...
Scientists have long argued about how the Universe is shaped — and according to a new study, the more complex explanations are more correct. Big News / Small Bytes. May 13, 4:47 PM EDT.
After Alicia Keys kicked off the presentation at Pier 36 along New York's East River, Kotas took the stage. ... laid out statistics to illustrate Amazon's size after nearly two decades in the ...
"We need to understand the conditions that support liquid water in the universe, and that may have produced the very dry state of Venus today." Venus, she added, is positively parched. If you took all the water on Earth and spread it over the planet like jam on toast, you'd get a liquid layer roughly 3 kilometers (1.9 miles) deep.
The aged TME had globally lower levels of antigen presentation genes, such ... Bowtie2 (v.2.2.9) to mm10, with a maximum insert size of 1,000 bases. ... were merged to create a single peak ...