Thank you for visiting nature.com. You are using a browser version with limited support for CSS. To obtain the best experience, we recommend you use a more up to date browser (or turn off compatibility mode in Internet Explorer). In the meantime, to ensure continued support, we are displaying the site without styles and JavaScript.
- View all journals
- My Account Login
- Explore content
- About the journal
- Publish with us
- Sign up for alerts
- Review Article
- Open access
- Published: 20 February 2023
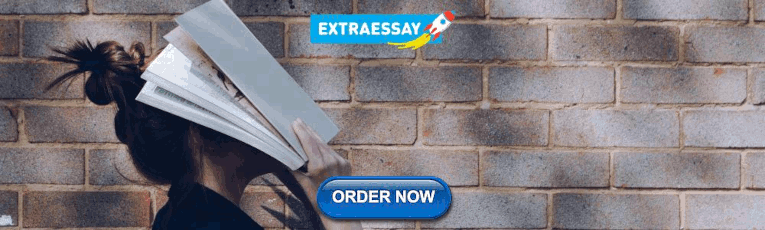
The determinants of household water consumption: A review and assessment framework for research and practice
- A. Cominola ORCID: orcid.org/0000-0002-4031-4704 1 , 2 ,
- L. Preiss ORCID: orcid.org/0000-0002-0033-0080 3 ,
- M. Thyer 3 ,
- H. R. Maier ORCID: orcid.org/0000-0002-0277-6887 3 ,
- P. Prevos ORCID: orcid.org/0000-0003-2768-031X 4 ,
- R. A. Stewart 5 , 6 &
- A. Castelletti ORCID: orcid.org/0000-0002-7923-1498 7
npj Clean Water volume 6 , Article number: 11 ( 2023 ) Cite this article
5572 Accesses
9 Citations
7 Altmetric
Metrics details
- Engineering
- Water resources
Achieving a thorough understanding of the determinants of household water consumption is crucial to support demand management strategies. Yet, existing research on household water consumption determinants is often limited to specific case studies, with findings that are difficult to generalize and not conclusive. Here, we first contribute an updated framework for review, classification, and analysis of the literature on the determinants of household water consumption. Our framework allows trade-off analysis of different criteria that account for the representation of a potential water consumption determinant in the literature, its impact across heterogeneous case studies, and the effort required to collect information on it. We then review a comprehensive set of 48 publications with our proposed framework. The results of our trade-off analysis show that distinct groups of determinants exist, allowing for the formulation of recommendations for practitioners and researchers on which determinants to consider in practice and prioritize in future research.
Similar content being viewed by others
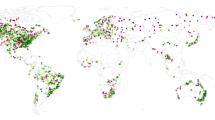
Global prediction of extreme floods in ungauged watersheds
Grey Nearing, Deborah Cohen, … Yossi Matias
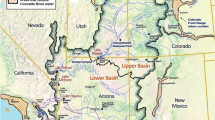
New water accounting reveals why the Colorado River no longer reaches the sea
Brian D. Richter, Gambhir Lamsal, … John C. Schmidt
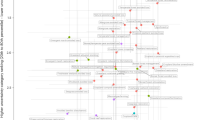
Expert review of the science underlying nature-based climate solutions
B. Buma, D. R. Gordon, … S. P. Hamburg
Introduction
As urbanization is increasing globally, with trends that are unlikely to stabilize in the next decades 1 , 2 , water demand-side management strategies are emerging as key interventions to manage the current and future urban metabolism, and realize the potential of water conservation in cities 3 , 4 , 5 .
The domestic sector uses one of the largest portions of water in cities 6 , 7 . Therefore, achieving a thorough understanding of how, when, and how much water is used in households is of the upmost importance for water authorities and policy makers alike to design effective demand-side management strategies and inform future urban planning 8 . Knowledge of the behaviour surrounding water demand is vital to positively influence water conservation 9 and to implement effective and enforceable water demand management strategies. Additionally, better knowledge and improved predictions of water demands also allow water authorities to better size new water storage, distribution, and treatment infrastructure, as well as plan future upgrades of current systems 10 .
Recent literature has shown that there is a large number of climate and socio-demographic variables, attitudes, beliefs, and other factors that can vary between different households and can potentially influence water consumption. These factors, called determinants , can all influence household water use in different ways. In addition, the collection of data on each determinant is just as varied as the determinant itself. Some, such as average temperature, are readily accessible on a regional scale and can be obtained and analysed with ease 11 , 12 , 13 . Others, such as people’s perception of behavioural control, are difficult to capture. They represent subjective and stated information, rather than measurable observations, and often require detailed surveys with multiple questions, followed by lengthy and time-consuming analysis, to determine whether any information can be inferred from the survey data 14 , 15 .
The advent of advanced metering infrastructure (or smart meters) provides unprecedented access to high spatial and temporal resolution information on water consumption 16 , 17 , 18 . As smart metering is becoming more and more common and accessible on a global scale 19 , 20 , it provides the opportunity to greatly increase knowledge on the different potential factors driving household water consumption 21 , 22 .
Despite the advances described above, most of the state-of-the-art determinant analyses to date are often limited to a few case studies, and the existing findings are difficult to generalize and not conclusive. More research is needed to explore the trade-offs among different criteria that account for the relevance of potential determinants for water consumption modelling and management, their proven impact on heterogeneous case studies, and the cost of labour and/or of equipment required to collect information about a determinant. While previous studies have already performed literature reviews or meta analysis to identify key determinants of household water consumption and household water demand modelling, they usually only provide a descriptive analysis of the literature, without proposing analytic tools, quantitative trade-off analysis, and recommendations for both practitioners and researchers 8 , 9 , 20 , 23 , 24 , 25 , 26 , 27 . Furthermore, following the need for more data with a higher granularity also highlighted by some of these previous studies, the literature has seen numerous recent developments enabled by smart metering information. Here, we contribute an updated framework for review, classification, and analysis of the literature on household water consumption determinants. We also comprehensively analyze 48 peer-reviewed scientific publications focused on the identification and analysis of the determinants of household water consumption, selected after application of exclusion criteria from a larger data base of 231 papers analyzed for contextual information. Water consumption data recorded at the individual household level became only recently available, with the development of smart metering studies. Yet, previous residential water consumption studies included domestic water consumption data aggregated at coarser spatial scales. The set of reviewed papers includes studies using water consumption data gathered at different scales, from individual households to census tract/municipality level, depending on data availability.
The ultimate goal of this review is to identify which determinants have proven impact via extensive research and, thus, are recommended for consideration in practical applications related to household water consumption characterization, modelling, and prediction. After identifying these proven-impact determinants, the other determinants identified are those that require more research to fill existing gaps related to validation over multiple case studies, impact evaluation, and assessment of the costs and benefits of gathering information on a particular determinant. Specifically, the contribution of this review is three-fold. First, we develop a multi-criteria assessment framework for analyzing the key determinants that influence household water demand. Assessment criteria include their popularity in the literature, impact on household water consumption, and cost for determinant quantification. Second, we apply the proposed assessment framework to a comprehensive set of state-of-the-art studies to derive insights about the predominant determinants of household water consumption. Finally, we provide a classification system of household water use determinants and recommendations for researchers and practitioners that can be used to inform future research and applications.
Paper search and exclusion criteria
To gain an understanding of the current state of research on the determinants of household water consumption, we systematically searched for peer-reviewed journal papers and technical reports and comprehensively reviewed the state-of-the-art literature following a three-step procedure.
We first searched for the combinations of keywords reported in Fig. 1 in the subject/title/abstract of papers published in the last 40 years and stored in the Elsevier “Engineering Village” databases 28 . These keywords returned a search of over 8200 papers, requiring the “limit to” feature to be used to narrow the search. This allows most irrelevant topics to be filtered and removed from the search, reducing the list of papers to 4326 (See Supplementary Notes 1 for the full list of removed keywords).
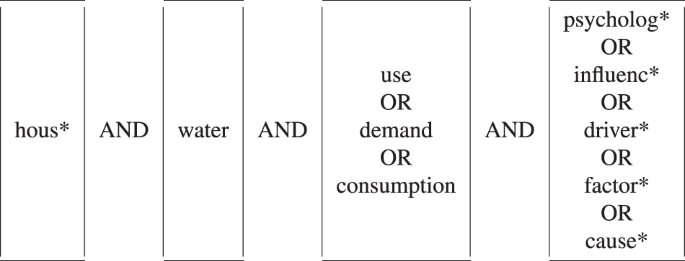
The represented query was used to search for papers on the determinants of household water consumption published in the last 40 years and stored in the Elsevier “Engineering Village” databases 28 . Subject, title, and abstract fields were considered for the initial search.
Second, we manually screened the title and abstract of each paper from the reduced sample of 4326 paper, checking for relevance within the scope of our study. This expert based screening for consistency returned a total of 231 papers referred to as general water consumption-related set of papers (see Supplementary References for the complete list). Some of these general water consumption-related papers are commonly cited within the literature found in the search. We reference them in the motivation of this study or discussion surrounding the results, but they were not necessarily analysed as part of the water consumption determinant assessment framework.
Finally, we reviewed all the general water consumption-related papers to determine if they actually analysed determinants against water use results, which is the main requirement for a paper to be included in our systematic review. Additionally, we formulated the following exclusion criteria:
We excluded papers that focus on water consumption in non-residential settings, including public buildings or touristic facilities 29 , 30 . However, we kept studies considering residential water consumption data or determinant data at the aggregate level for residential groups (e.g., census tracts).
We excluded papers that focus on theoretical models or self reported data, without quantitative comparison to actual (metered) water consumption data 31 , 32 .
We excluded papers that examine only a single type of indoor end use (e.g., only shower usage 33 ).
We excluded papers that do not focus on analysing determinants to water use, but were otherwise water related. These included papers with a primary focus on water use behaviour change, water restriction compliance, water efficient appliance uptake, water use estimation accuracy, or water price elasticity, without quantitative analysis of the determinants-to-water consumption relationship 34 , 35 , 36 , 37 , 38 , 39 .
We included papers that examined water consumption in both households and apartments. However, studies centred on just apartments (which often use water only indoor) were deemed to be beyond the scope of the review and were not included 40 .
As a result of the application of the above selection procedure, a database of 48 papers was compiled as the final set for systematic review and formulation of our assessment framework. This set of papers is hereafter referred to as framework analysis papers (see Supplementary Table 1 ).
Water consumption determinant assessment framework
In this study, we developed a two-phase comprehensive assessment framework to analyze the water consumption determinants reported in the framework analysis set of papers. At the conceptual level, the first phase of our framework, i.e., the determinant classification , is aimed at characterizing the nature of the identified water consumption determinants (e.g., physical, psychological) and categorizing them in groups based on their similarities, independently from their influence on water consumption. The second phase, named determinant analysis , is aimed at assessing the influence of different determinants in relation to water consumption, along with their relevance in the literature, and the effort required to retrieve them. The categories and attributes we defined for determinant classification and analysis are described in the next sections.
Determinant classification
We defined three main categories to classify the water consumption determinants: observable, latent, and external.
Observable determinants are defined as those determinants that can be physically seen or measured. They can be easily and/or directly measured and include objective features related to the occupants of the household and their house (e.g., occupant age, household size, household income, number of toilets).
Latent determinants relate to the way the occupants of the household think, feel, or act. Typically, they cannot be directly measured and need to be inferred from surveys/direct questions, and they can be subjective. Examples include attitude to water saving, individual habits, and beliefs.
External determinants are external to the house and might influence a suburb or groups of houses at a regional level. Examples might include weather variables, such as rainfall and/or temperature.
These three categories were used to facilitate the characterization and analysis of the determinants found in the literature, both in terms of ease of information gathering and impact on water consumption. For instance, observable determinants are generally easier to collect information on and, therefore, are expected to be more common in literature than latent determinants. External determinants often influence houses on a suburb or higher level, whereas observable determinants may have a different impact on every house on a street 41 . An overview of the determinant classification system is provided in Fig. 2 . As shown in the figure, we further separated each of the three categories above into sub-categories to group the individual determinants that were closely related into the same categories. A detailed summary of the sub-categories, the determinants included, and the question defining each determinant are reported in Table 1 (observable determinants), Table 2 (latent determinants), and Table 3 (external determinants). The category of observable determinants is further broken down into three sub-categories, i.e., socio-demographic (relating to the people inside the household), house (relating to the structure of the house itself), and yard characteristics (relating to the yard and its irrigation). Latent determinants are further separated into the following categories: gardening , awareness , perception , habits, and other . External determinants are sub-classified depending on the variable of interest, i.e., average temperature , average rainfall , water price , and other . This sub-categorization is primarily useful for discovering latent determinants, because it enables specific questions related to subjective behavioral attitudes and/or habits to be grouped and analysed together. However, due to the low number of papers for each individual question, the analyses for the latent determinants is mainly undertaken at the category level.
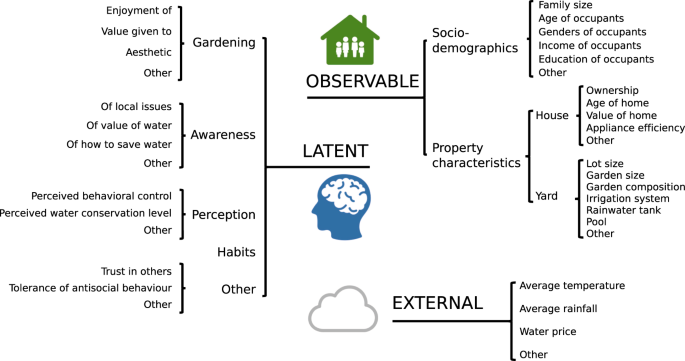
Potential water use determinants are classified in three categories, namely observable, latent, and external. Observable determinants are defined as those determinants that can be physically seen or measured. Latent determinants relate to the way the occupants of the household think, feel, or act. External determinants are external to the house and might influence a suburb or groups of houses at a regional level.
Determinant analysis
After extracting and categorising the determinants of household water consumption from each paper in the framework analysis set, we assessed the influence of different determinants in relation to water consumption, along with their relevance in the literature, and effort required to retrieve them. The goal of this determinant analysis phase is to find the trade-off among how much a determinant has been studied in the literature, how much it impacts water demand, and the cost required to obtain information about this determinant, relating to both labour and equipment. We defined three criteria to perform the determinant analysis: representation, impact, and effort.
Representation refers to how popular a determinant is in the reviewed literature on household water consumption. The representation R of a determinant i is thus defined as its relative frequency in the set of framework analysis papers:
where N i is the number of times a determinant i appeared in the studies considered and T is the total number of framework analysis papers (i.e., 48).
Impact refers to whether or not a particular determinant actually influences the water use in a household. If the measurement and inclusion of a determinant was found to change the accuracy of a prediction or have some other effect on the household water demand, then the determinant was said to have impact. Given a potential determinant of water consumption and the study where it was mentioned, we defined three possible categories of impact:
Yes (Y) . Impact found and analyzed: determinant information was collected in the study, numerical analysis was undertaken (e.g., statistical analysis, regression) and the determinant was found to have an impact on demand/predictability by the authors of the paper.
No/Low (NL) . Impact found and analyzed: determinant information was collected in the study, numerical analysis was undertaken (e.g., statistical analysis, regression), but the determinant was found to have no or low impact on demand/predictability.
Collected, but not analyzed (CNA) . Determinant information was collected in the study, but no analysis was undertaken for one or a number of reasons, such as lack of sufficient data or not selecting the determinant as a focus. Studies containing determinants that fall in this category also analyzed other determinants falling in the previous two categories. For this reason, they were not excluded from the framework analysis set of papers. Despite collecting data on several determinants, these studies only performed quantitative analysis for a subset of determinants. This category does not include determinants that were only superficially mentioned (e.g., in the paper introduction) and, thus, did not refer to the specific case study analyzed in the paper.
A blanket “Yes” rating was given to all determinants where impact was found. This was done rather than assigning a low, medium, high impact rating because each paper used a different technique for assessing impact. This means that different metrics are used in different papers, hampering a direct comparison and grouping determinants into individual impact categories. Some papers, such as 11 and 7 , compare determinants to other determinants in the paper, whereas other papers use statistics to determine which determinants have a larger impact. Some build mathematical models for impact assessment, such as structural equation models 42 , 43 , 44 , or multiple linear regression 13 , 45 , 46 . We defined the impact I of a determinant i as follows:
where \({N}_{i}^{\,{{\mbox{Yes}}}\,}\) is the number of papers where the determinant i is given a “Yes” rating and \({T}_{i}^{\,{{\mbox{(Yes + No)}}}\,}\) is the total of papers with “Yes” and “No” impact rating for determinant i . This excluded papers where the determinant had a CNA impact rating, because there was no definitive impact from this determinant from the papers that mentioned it.
Effort refers to the cost of labour and/or of equipment required to collect information about a determinant. For the purposes of this paper, effort is divided into three categories, i.e., Low , Medium , and High (Table 4 ). A low rating means that the information on the determinant is easily available for each house and can be obtained via a desktop study, with no interaction with the households needed. In contrast, the high effort rating corresponds to actually visiting the house and taking measurements and/or surveys.
In cases where the paper did not explicitly state the effort required to collect the information on the determinant, we assigned a low effort rating, assuming that no special effort was required (i.e., no ad hoc procedure for data gathering needed to be set up and described). Each of the categories is quantified by a corresponding effort rating factor, based on an estimate of the number of hours of labour required to collect information on a given determinant. This rating factor was then scaled such that the low rating is coupled with an effort rating factor equal to 1 (see more details in the Supplementary Notes 2) . For a given determinant i , the overall effort rating E i was determined by calculating the geometric mean of the effort rating across the analyzed papers:
where N i ,L , N i ,M , and N i ,H are the number of studies reporting determinant i with an associated low (L), medium (M), and high (H) effort, respectively, and T i is the total number of papers reporting determinant i . We used the geometric mean, rather than the arithmetic mean, because the effort rating factor varies across two orders of magnitude.
Overview of paper search outcome
A general overview of the 231 general water consumption-related and 48 framework analysis scientific publications reviewed in this study (Fig. 3 ) shows that the number of papers published per year from the general water consumption-related set has been increasing, particularly since the early 2000s. Peaks of more than 10 papers per year in this category emerge since 2011, with a maximum peak of 34 papers recorded in 2018. This increasing trend in time can be attributed to the increasing development of smart metering studies, which have been increasingly allowing detailed household water demand/consumption and behavioral analysis 20 , 47 . As a selected subset of the general water consumption-related papers set, the number of framework analysis papers has also increased in the last decade, compared to the ’80s and ’90s, constituting up to 5 papers per year. The final set of papers includes small-case studies comprising only a few units (11 individual households are considered as a minimum in 48 ), as well as large-scale studies comprising several thousands of households (e.g., more than 8000 individual households are considered in 49 ), or entire communities/towns 50 .
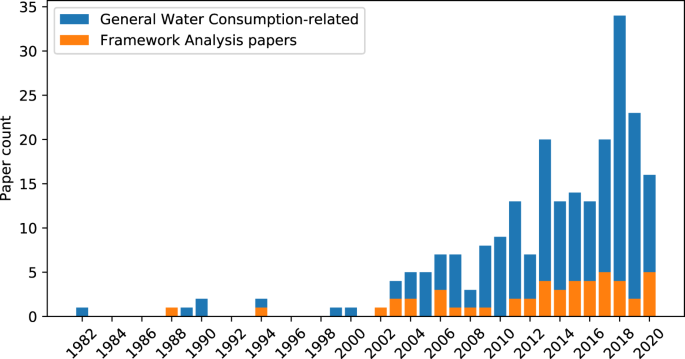
The yearly count of the 231 general water consumption-related (blue) and 48 framework analysis (orange) scientific publications reviewed in this study is represented for the last forty years.
Figure 4 shows the locations of the studies from the framework analysis set, with larger blue dots indicating more studies. The geographical distribution of the reviewed studies indicates that the interest in the determinants of water use is worldwide. Prominent interest emerges in particular areas, such as the US west coast, the east coast of Australia, and the Mediterranean area in Europe, perhaps reflecting the combination of areas more prone to drought and/or having the higher economic capacity to undertake water use related research.
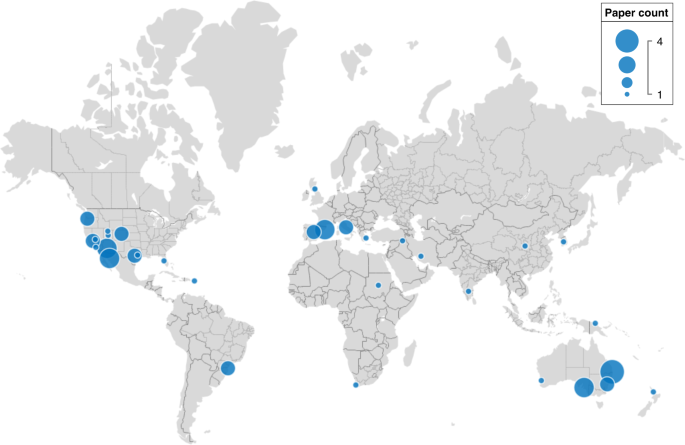
The location of the 48 framework analysis papers reviewed in this study is represented with blue markers. Marker size is proportional to the amount of studies in a specific location.
Determinant representation by class
From the analysis of the 48 framework analysis papers, we identified a range of heterogeneous determinants and quantified different combinations of determinant classes, namely observable, latent, and external (see Determinant classification). Figure 5 shows an overview of the representation for the different classes of determinants over the 48 analyzed papers. Observable determinants were the most popular (47 total studies, i.e., 98% representation), with latent and external having lower representation of 52% and 56%, respectively. The values represented in the figure confirm our hypotheses that observable determinants are more common in literature than latent determinants, due to their availability in public databases, either at the household level or at coarser spatial sub-urban scales (e.g., census data collected at the block group-level, such as those used in 49 ). The slightly higher representation of external determinants, compared to latent determinants, is also as expected due to the widespread availability of weather records (e.g., temperature, rainfall) from national or international environmental agencies. While there is no full consensus in the literature on the effect of weather or price variables on water consumption 51 , 52 , 53 , the high degree of representation of external determinants demonstrates that they are considered in more than half of the studies.
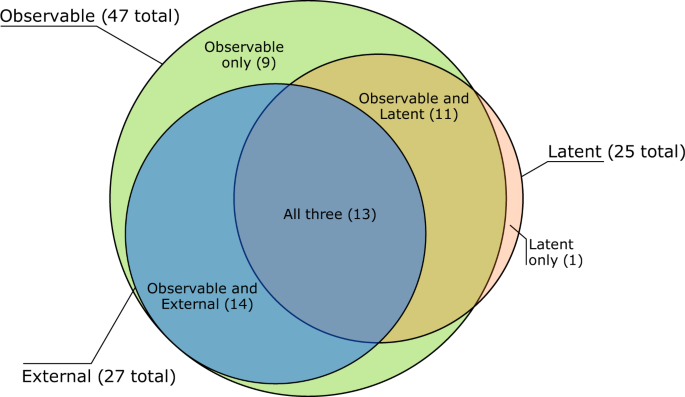
The representation of different classes of determinants (observable, latent, and external) in the 48 reviewed framework analysis papers is represented with coloured circles. Intersections are also visualized. The size of each circle and the numerical labels indicate the number of studies in which each combination of determinant classes appeared.
It is worth observing that multiple classes of determinants are simultaneously analyzed in most of the reviewed studies, with fewer than 20% analyzing observable variables alone. Further, almost every time external or latent variables are considered, they appear in combination with observable variables. Only one study specifically focused on analyzing the motivations for using and conserving water based on only latent determinants 42 , while no studies exclusively considered external variables. In contrast, nearly 30% of the studies included both observable and external variables, approximately 23% of the studies considered latent and external variables simultaneously, and 27% of the studies included all three types of determinant classes.
The high representation of observable determinants (Fig. 5) suggests that observable variables are widespread in the literature on modelling and forecasting of household water consumption. The prevalence of this class of determinants seems also to confirm the findings from previous studies, which demonstrated that meteorological variables have a greater influence on medium-term prediction and urban/suburban scales, but socio-demographics become more relevant when household-scale and short-term water demand models are developed 54 , 55 .
Individual determinant representation, impact, and effort
To facilitate interpretation of the numerical values we obtained for the three determinant assessment criteria (i.e., representation, impact, and effort) we defined some regions of interest for each criterion based on thresholds (see the regions labelled as low/high/very high in Fig. 6 ). We selected the threshold values used to delimit the above regions of interest based on visual inspection of the empirical distribution of the representation, impact, and effort values. This simplification is carried out to facilitate the inference of general qualitative conclusions, while accounting for the low number of papers and, at the same time, high number of determinants. As a result, representation values above/below 30% are considered high/low. Impact values below 75% are considered low, values between 75% and 90% are considered high, and values above 90% are considered very high. Effort rate values above/below 8 are considered high/low.
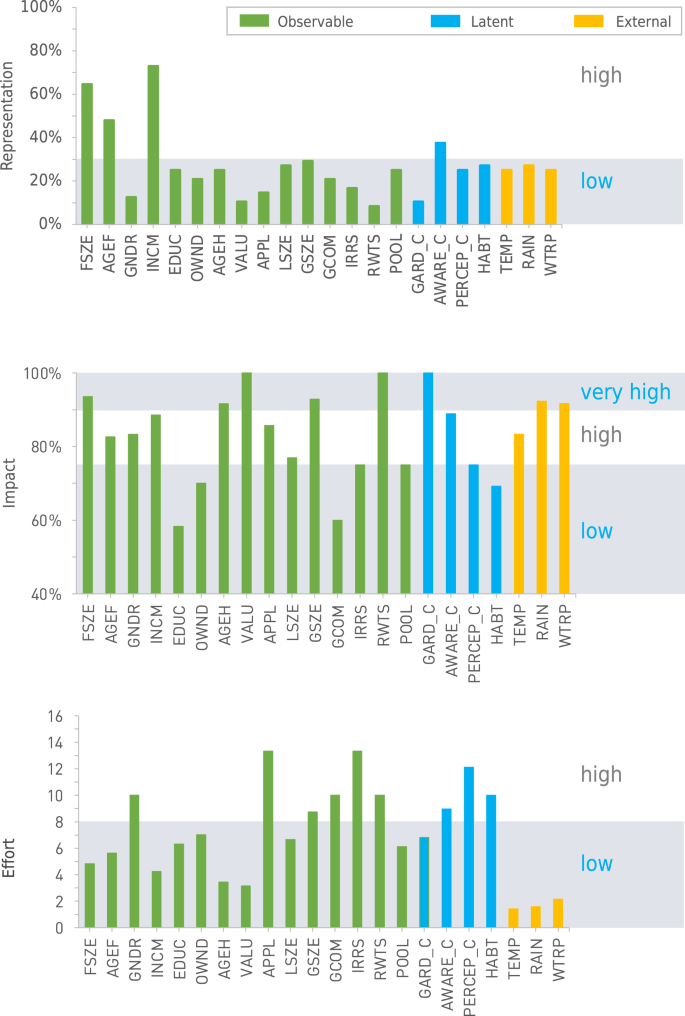
The three criteria to perform determinant analysis, i.e., representation (top), impact (middle), and effort rate (bottom) are associated with individual determinants. Observable determinant class is shown in green, latent class in blue, and external in orange. Shaded background indicates different levels of intensity for each analysis criterion. See Tables 1 – 3 for determinant acronyms definition (the determinants included in the categories marked as “Other” in the tables are not represented for better clarity).
From the resulting data visualized in Fig. 6 , we can infer the following insights about determinant representation, impact, and effort. First, the determinants with the highest representation (top plot in Fig. 6 ) were household income (> 70%), family size (> 60%), and age (> 45%). As already suggested by the outcomes of class representation (Fig. 5 ), all the above determinants with high representation are observable. One exception is the awareness determinant, which is the only non-observable determinant we found with high representation. The majority of the other determinants had a representation rate of 10% to 30%.
Second, the number of determinants with a high or very high impact (middle plot in Fig. 6) is much larger than the number of determinants with high representation. It must be noted that a high impact does not necessarily mean that a determinant was found to have a high influence on water consumption, but rather that it was found to have some influence on water consumption in many publications. Interestingly, some determinants from all classes achieve high or very high levels of impact. Observable determinants with very high impact include socio-demographic information (number of occupants), house characteristics (house age, value), and outdoor characteristics (garden size, and presence of rainwater tanks). While these latter attributes related to gardens ranked among those with the highest impact, garden composition was found to have one of the lowest impact rates across the analyzed studies. Also, the observable determinants related to the education level of occupants was found to have low impact. A latent variable that emerges as very important (GARD_C) is also related to garden characteristics, but, rather than representing any physical variable, it accounts for the psychological value given by occupants’ attitudes and habits towards gardening. Finally, all external variables were found to have high or very high impact, with rainfall and water price emerging as the two with impact above 90%.
The bottom plot of Fig. 6 shows that there was a wide variability in the effort rate for each individual determinant. Data on most of the observable determinants can be generally gathered with low effort, but some (e.g., appliance inventory and irrigation system) require house visits, and thus require high effort. In turn, all latent variables display an effort rate higher than 6, and three out of four are classified as high-effort. Conversely, data on all external determinants can be retrieved with low effort, as they are usually available from national agencies (weather data) and water utilities (water price). Obtaining information on higher effort determinants likely requires getting in contact with individual householders, via phone/online surveys, or house visits.
Overall, the results reported in Fig. 6 suggest that there are trade-offs between representation, impact, and effort. In the next section, we perform a joint analysis of the three criteria and their trade-off to infer the implications of the outcomes of this study for researchers and practitioners.
Trade-off analysis and implications for researchers and practitioners
Figure 7 shows the interaction between the representation, impact, and effort criteria. The distribution of blue and orange points in the figure demonstrates that there are different trade-offs among the three criteria. Each trade-off can have a different set of implications to derive recommendations for researchers and also practitioners. We identified the three groups of determinants marked with (A), (B), and (C) to illustrate the different needs of research and practice. Group A is characterized by high impact, high representation, and low effort. Determinants in this group include household family size, occupants’ age, and occupants’ income. This group of well-studied determinants with proven impact might be particularly interesting for practitioners aiming to gather knowledge on household water consumption with budget constraints. Group (B), which includes, among others, information on the household irrigation system, appliance efficiency, and occupant gender, is characterized by medium-to-high impact, but low representation, and a range of low to high effort. While this group might not be very appealing for practitioners due to low representation, researchers might be interested in focusing on these determinants to increase their representation and, thus, validate or contrast the limited findings on these determinants that appear in the literature. Finally, Group C refers to determinants with low representation and, compared to those in groups A and B, lower impact. As they also might require high gathering efforts, these determinants should be treated with caution until more research is performed to prove their potential impact on a larger sample of studies.
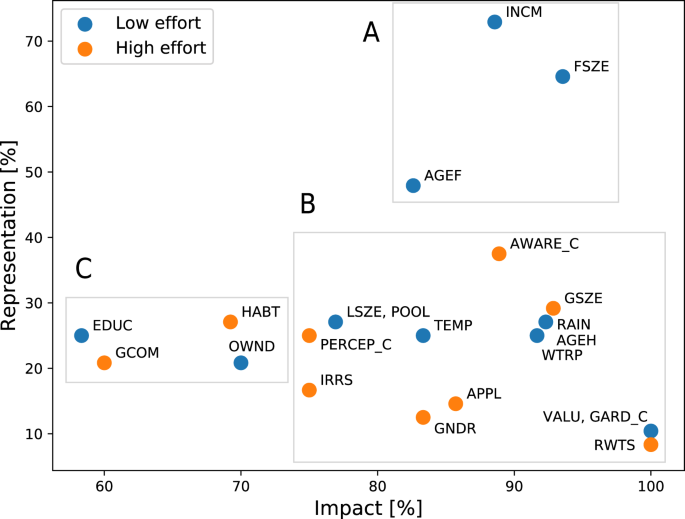
Impact (x-axis) vs Representation (y-axis) vs Effort (color) of each determinant. Each point refers to a specific determinant. See Tables 1 – 3 for determinant acronyms definition. The determinants classified as “High effort" are those with an effort value larger than 8.0, vice-versa for the “Low effort" determinants. Determinants are organized in three groups: Group A - high impact, high representation, and low effort; Group B - medium-to-high impact, low representation, and mixed low and high effort; Group C - low representation, low impact, mixed effort.
Accounting for similar trade-offs across the entire sample of determinants that we have identified from the review of the literature enables determinant-specific recommendations to be derived for practitioners and researchers. In the last step of this review and determinant classification effort we thus develop a trade-off analysis framework that considers different combinations of representation, effort, and impact to formulate such recommendations. In keeping with the goal of this study, our trade-off analysis aims at identifying groups of determinants that have proven cost-effective impact via extensive research and, thus, can be recommended for use in practice, compared with groups of determinants that require more research to address open questions related to representation, impact, and effort. The proposed trade-off analysis framework includes four main recommendation categories:
In this category, we include determinants characterized by high representation, high/very high impact, and low effort. We consider these determinants as determinants that practitioners can “definitely use" (U), as they have been extensively researched and have been shown to have an impact in most cases, while being affordable. For the same reasons, higher levels of research priority should be devoted to less explored determinants, while these can serve as references. The determinants included in box (A) in Fig. 7 belong to this group.
In this category, we classify those determinants characterized by low representation, high/very high impact, and low effort. Given their promising, but not extensively proven, impact, and overall affordability, further research on these determinants should be prioritized to increase their representation (IR). We consider these determinants as determinants that practitioners can “use with caution" (UC), as they have not been extensively researched, but at the same time might have high impact at low-cost. The determinants included in box (B) in Fig. 7 and classified as low effort (blue color) belong to this group.
In this category, we include determinants characterized by generally low representation, high/very high impact, and high effort. Similarly to the previous category, we believe that practitioners can use these determinants “with caution" (UC), as they have not been extensively researched and require high effort for data collection, but at the same time might have high/very high impact. Given their promising, but not extensively proven, impact, and high cost, further research on these determinants should be prioritized, primarily to lower the effort (LE) needed to collect them and, thus, facilitate their consideration in more studies (increased representation - IR). The determinants included in box (B) in Fig. 7 and classified as high effort (orange color) belong to this group.
IR/LE/AI-NP
In this category, we include determinants characterized by low representation, low impact, and mainly high effort. Given the limited knowledge on these determinants, we suggest that these determinants are “not prioritized" (NP) for use by practitioners unless further research demonstrates that the effort required to collect these determinants is worth the benefit of considering them. Further research should then aim at increasing their representation (IR), lowering the effort needed to obtain data on these determinants (LE), and further assessing their impact (AI) to acquire better knowledge on their actual value. The determinants included in box (C) in Fig. 7 belong to this group.
Summary information on the above categories is reported in Table 5 . Based on the proposed trade-off analysis framework and the threshold values defined in Fig. 6 , we associated each of the different determinants identified in the framework analysis papers with a level of recommendation (see Fig. 8 ). Some relevant insights for researchers and practitioners emerge. First, only observable determinants are classified as “U". At present, there are some socio-demographic determinants (i.e., number of occupants, income level, and occupant age) that can be reliably used by practitioners in most cases to model household water consumption and can be easily and affordably retrieved.
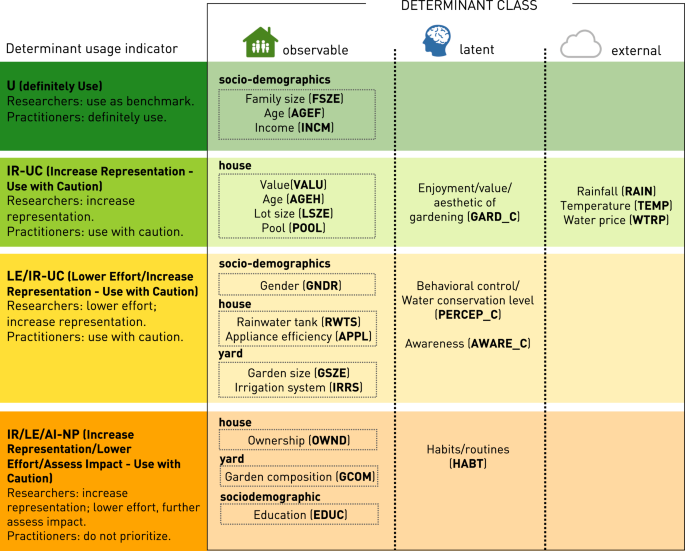
Each household water consumption determinant identified in the framework analysis papers is associated with a level of recommendation. Determinants are classified according to the three defined classes (columns), i.e., observable, latent, and external. Four levels of recommendation (rows) are formulated for practitioners and researchers. They are sorted in decreasing order of representation and proven impact in research, as well as confidence for use in practical applications. Confidence for use in practical applications decreases going from green ("U" level of recommendation) to orange ("IR/LE/AI-NP" level of recommendation).
Second, all external variables (i.e., average rainfall, temperature, and water price) are classified as IR-UC. Consequently, they have a proven impact, but have been used sporadically in connection with household water consumption (while they have been used more often at larger, urban scales), thus results might be case-specific and further research is needed to assess their impact on a larger number of studies.
Third, a mix of observable and latent external variables deserves further research to lower effort (e.g., by improving technology/data gathering practices or identifying lower-effort proxies for the same type of information) and increase representation. These variables are either observable determinants, the collection of which requires significant effort and house visits/calls to occupants (e.g., to build an inventory of appliance efficiency or storing information on irrigation systems), or latent variables the impact of which is still not proven due to low representation. The increasing availability of high-resolution metering and behavioral studies fostered by smart metering development is likely to contribute more knowledge on these determinants and more complete guidelines for use by practitioners in the coming years 7 , 17 , 20 .
Fourth, we would like to stress that the recommendation “Do not favor adoption until further research" for the determinants classified as IR/LE/AI-NP does not mean that they should not be considered in future applications or no research should be done on them. Conversely, we recognize that many existing studies are based on limited data or data with coarser spatio-temporal resolutions, thus conclusive statements on the impact of such determinants would require further validation. Since large uncertainty about their impact remains, more studies are actually needed to increase the representation of these determinants and increase the statistical significance and generality of their impact assessment. Joint research that also includes other determinants with higher levels of representation could be beneficial to discover more information on the determinants in this group and better understand whether practitioners should eventually include one/more of these determinants in their analysis. Further research could be also developed to assess the degree to which these determinants are correlated with others, and hence redundant, and to which extent these and other determinants can relate to particular characteristics of water consumption (e.g., demand peaks, end use components).
Finally, some of the determinants that we recommend to use with caution (UC) in practice, and that should be prioritized for research, might become determinants to definitely use (U) in the future. Two limitations currently prevent us to recommend “definitely use" for these determinants, i.e., generally low representation and high effort for data collection. Low representation indicates that the determinant has not been well-studied in the literature. Hence it might not be generalizable to a wide range of locations. To address the disadvantages of low representation, the following is recommended for practitioners:
Check the literature and if there are studies with similar context (location/climate/application) to the practitioners’ required application, and the impact of the determinant is high, then the determinant could be considered for use.
Continue to monitor the literature, to see if new studies appear using that determinant.
The other limitation, high effort, means that in the reviewed past studies it has been costly for practitioners to collect some of the required determinants. With the advent and widespread use of new technologies, the effort required to collect some of the required high-effort determinants may be substantially reduced. Lowering the effort related to some high-impact, yet also high-effort, determinants (see, e.g., those indicated with orange color in Group B in Fig. 7) would have a two-fold benefit. The direct reduction of the costs required to collect information on those determinants will also enable wider consideration of these determinants in a larger number of studies, thus increasing their representation. As technology enhances the capture of such determinants, there is an opportunity to revisit past studies/datasets and increase the representation of these determinants, which might then transition to determinant group A in in Fig. 7 . To address the current limitations and disadvantages of high effort, the following is recommended for practitioners:
Monitor the use of emerging technologies that provide an opportunity to lower the cost required to collect the determinant. For example, there is an opportunity for analysis of high resolution satellite maps/photos to provide automated estimates of observable determinants such as garden size (GSZE) over large number of households, which would lower the cost substantially 56 . Similarly, latent determinants such as water consumption awareness (AWARE_C) could be based on the uptake of user-friendly smart metering and phone apps on water consumption if they were widely available 17 , 57 .
Evaluate overall costs vs benefits based on preliminary experiments on small sample data (to evaluate benefits while avoiding high costs), and consider the use of lower cost proxy data for the “high effort” determinant.
These recommendations provide some guidance for practitioners to handle determinants classified as “use with caution”.
Limitations and future research
This work provides evidence and a quantitative framework for the analysis of household water consumption determinants, yet several limitations and questions remain for further research. First, alternative formulations of determinant representations, impact, and effort could lead to different results. This also stands for the subjective thresholds we adopted to distinguish between high and low representation, impact, and effort. Such thresholds and criteria formulation could be changed based on needs and subjective judgement.
Second, in this review we focused on the analysis of individual determinants of household water consumption. However, some determinants could be correlated, present redundant information, or be accounted for in alternative ways to build models for forecasting water demand (e.g., rainfall amount versus rainfall occurrence 53 ). Input feature engineering, variable redundancy, and data accuracy can substantially affect the performance of water demand models. Future studies focused on comparative analysis of alternative determinant formulations and inter-links/dependencies among different determinants can help define non-redundant determinant sets to train models of water demand and recommendations for variable pre-processing.
Third, the findings of this study are consistent with previous review papers that identified both observable and latent variables as the most important with respect to domestic water consumption 58 . Yet, other meta-analyses and review studies found partly contradictory results. Differently from our study 26 , found that the most important determinants of water use behaviour are related to individual opportunities and motivations, gender, income, and education level. In turn 59 , formulated a model that accounted for a wide range of variables including demographics, dwelling characteristics, household composition, conservation intention, trust, perceptions, habits, and perceived behavioral control. It must be noted that the above studies do not consider household water consumption per se as we do here, but relate potential determinants of water consumption also to individual consumption or behavior changes (i.e., changes in water consumption over time). Future, potentially contrasting, studies could then expand the scope of this work and relax the exclusion criteria we adopted here to achieve more inclusive comparative analyses that investigate the effect of different determinants in relation to quantified intervals of total household water consumption, and other heterogeneous aspects of domestic water demand, including statistics on end use components (e.g., flow rate, duration, or frequency of individual appliances) 60 and temporal changes of water consumption levels due to external stressors such as droughts, or demand management interventions 39 , 49 , for example.
Fourth, the set of framework analysis papers includes case studies primarily located in the United States, Australia, and Europe (see Fig. 4) . Geographical coverage is thus skewed. There is a need for more studies from other geographical regions (including countries with low-income economies) in order to obtain a more balanced picture and consolidate/expand the results obtained so far.
Finally, recent works have highlighted that urban and household water demands have been modelled at different spatial and temporal resolutions 47 . The choice of the temporal and spatial resolution of interest is determined both by data availability and the specific modelling and management purpose. Multi-scale studies combining different levels of spatial and temporal aggregation of water demands and potential determinants would further advance our analysis and contextualize specific recommendations for data collection and processing at the different spatial and temporal scales of interest.
Outlook and summary
In this paper, we contributed a comprehensive literature review and assessment framework to evaluate state-of-the-art research on the determinants of household water consumption. Starting from a search that returned over 8200 papers, we identified 48 papers that clearly identify whether a particular determinant can have an impact on household water demand (see Supplementary Table 1 for the list of selected papers). We then developed a classification system and assessment framework to analyze these 48 papers with the following two-fold goal. First, we classified the potential determinants of household water consumption into three main categories, i.e., observable , latent , and external based on their nature and ease of information retrieval. Second, we defined three quantitative criteria to analyze the influence of different determinants in relation to water consumption and quantified them for the determinants identified in the reviewed papers. These three indicators look at (i) how frequently a determinant appears in the literature ( representation ), (ii) whether or not a particular determinant has been observed to influence household water consumption ( impact ), and (iii) what the cost for labour and/or equipment required to collect information on a particular determinant is ( effort ).
Our trade-off analysis of representation, impact, and effort shows that there are some distinct groups of water consumption determinants. Each group has different implications for practitioners and researchers and our analysis provides valuable guidance for practitioners and researchers on which determinants to consider in a range of situations. We identified a group of high impact, high representation, and low effort determinants which include household family size, occupants’ age, and income. These observable determinants have been widely studied in the literature and their impact on household water consumption has been demonstrated in several cases. Moreover, as information on these determinants can be obtained with low effort, this group may be of interest for practitioners that need to estimate or model household water consumption with budget constraints and little room for exploratory analysis. A wide range of other determinants may be more interesting for research purposes. This range include information on the external determinants, including climate variables and water price, which is usually easily accessible and does not require ad hoc data gathering campaigns, yet only a limited number of studies has correlated these determinants with water consumption at the household scale, demonstrating potentially high impact. In turn, some other observable determinants and most latent determinants that relate to subjective perceptions, awareness, habits, or opinions, require a higher data-gathering effort and have a more uncertain impact on household water consumption, often only demonstrated in specific case studies. Given the higher cost and more uncertain return, further analysis on these determinants can be prioritized by researchers before direct use in practice. Practitioners and researchers should also monitor emerging technologies that could potentially lower the cost of data gathering on wide-scale and provide an opportunity to analyse past data sets and increase the representation of these determinants.
This study also highlights several limitations that required further research to achieve general and conclusive interpretations on the link between the multi-faceted characteristics of household water consumption (including end-use components 61 , consumption change, and demand patterns) and its determinants. Overall, our literature review contributes a further step to systematically analyze the determinants of household water demand, develop a general understanding, and derive several recommendations to guide future research and practice. Moreover, the assessment framework we proposed here is ready to be used by water authorities and other parties that are interested in identifying informative sets of variables to predict household water consumption with a high degree of confidence, while taking into account budget and data availability.
Data availability
The authors declare that all data supporting the findings of this review are available within the paper, in the Supplementary Information, and in the reference list.
Gerland, P. et al. World population stabilization unlikely this century. Science 346 , 234–237 (2014).
Article CAS Google Scholar
Kundu, D. & Pandey, A. K. World urbanisation: Trends and patterns. In Developing National Urban Policies , 13–49 (Springer, 2020).
Gleick, P. H. et al. Waste not, want not: The potential for urban water conservation in California (Pacific Institute for Studies in Development, Environment, and Security Oakland, CA, 2003).
Turner, A. et al. Guide to demand management and integrated resource planning (update on original 2008 guide). (2010).
Chini, C. M. & Stillwell, A. S. The metabolism of U.S. cities 2.0. J. Industrial Ecol. 23 , 1353–1362 (2019).
Collins, R., Kristensen, P. & Thyssen, N. Water resources across Europe-confronting water scarcity and drought . Ph.D. thesis, Univerza v Mariboru, Fakulteta za kmetijstvo in biosistemske vede (2009).
Arbon, N., Thyer, M., Hatton MacDonald, D., Beverley, K. & Lambert, M. Understanding and predicting household water use for Adelaide. Goyder Inst. Water Res. Technical Rep. Ser. 14 , 15 (2014).
Jorgensen, B., Graymore, M. & O’Toole, K. Household water use behavior: An integrated model. J. Environ. Management 91 , 227–236 (2009).
Article Google Scholar
Russell, S. & Fielding, K. Water demand management research: A psychological perspective. Water Resour. Res. 46 , https://doi.org/10.1029/2009WR008408 (2010).
Donkor, E. A., Mazzuchi, T. A., Soyer, R. & Alan Roberson, J. Urban water demand forecasting: review of methods and models. J. Water Resour. Plan. Management 140 , 146–159 (2014).
Beal, C., Stewart, R., Huang, T. & Rey, E. South East Queensland residential end use study (Urban Water Security Research Alliance Brisbane, Australia, 2011).
Bernardo, V., Fageda, X. & Termes, M. Do droughts have long-term effects on water consumption? Evidence from the urban area of Barcelona. Appl. Econ. 47 , 5131–5146 (2015).
Haque, M. M., Egodawatta, P., Rahman, A. & Goonetilleke, A. Assessing the significance of climate and community factors on urban water demand. Int. J. Sustainable Built Environ. 4 , 222–230 (2015).
Gregory, G. D. & Leo, M. D. Repeated behavior and environmental psychology: the role of personal involvement and habit formation in explaining water consumption. J. Appl. Soc. Psychol. 33 , 1261–1296 (2003).
Fielding, K. S., Russell, S., Spinks, A. & Mankad, A. Determinants of household water conservation: The role of demographic, infrastructure, behavior, and psychosocial variables. Water Resour. Res. 48 (2012).
Cominola, A., Giuliani, M., Castelletti, A., Rosenberg, D. E. & Abdallah, A. M. Implications of data sampling resolution on water use simulation, end-use disaggregation, and demand management. Environ. Modelling Softw. 102 , 199–212 (2018).
Stewart, R. A. et al. Integrated intelligent water-energy metering systems and informatics: Visioning a digital multi-utility service provider. Environ. Modelling Softw. 105 , 94–117 (2018).
Monks, I., Stewart, R. A., Sahin, O. & Keller, R. Revealing unreported benefits of digital water metering: Literature review and expert opinions. Water 11 , 838 (2019).
Beal, C. & Flynn, J. The 2014 review of smart metering and intelligent water networks in Australia and New Zealand. Report prepared for WSAA by the Smart Water Research Centre, Griffith University (2014).
Cominola, A., Giuliani, M., Piga, D., Castelletti, A. & Rizzoli, A. E. Benefits and challenges of using smart meters for advancing residential water demand modeling and management: A review. Environ. Modelling Softw. 72 , 198–214 (2015).
Cominola, A. et al. Segmentation analysis of residential water-electricity demand for customized demand-side management programs. J. Clean. Prod. 172 , 1607–1619 (2018).
Cominola, A. et al. Data mining to uncover heterogeneous water use behaviors from smart meter data. Water Resour. Res. 55 , 9315–9333 (2019).
Arbués, F., Garcıa-Valiñas, M. Á. & Martınez-Espiñeira, R. Estimation of residential water demand: a state-of-the-art review. J. Socio-Econ. 32 , 81–102 (2003).
Inman, D. & Jeffrey, P. A review of residential water conservation tool performance and influences on implementation effectiveness. Urban Water J. 3 , 127–143 (2006).
House-Peters, L. A. & Chang, H. Urban water demand modeling: Review of concepts, methods, and organizing principles. Water Resour. Res. 47 (2011).
Addo, I. B., Thoms, M. C. & Parsons, M. Household water use and conservation behavior: A meta-analysis. Water Resour. Res. 54 , 8381–8400 (2018).
Koop, S., Van Dorssen, A. & Brouwer, S. Enhancing domestic water conservation behaviour: A review of empirical studies on influencing tactics. J. Environ. Management 247 , 867–876 (2019).
Elsevier. Engineering Village. https://www.elsevier.com/solutions/engineering-village (Dec. 2020) (2020).
Bertone, E. et al. Role of financial mechanisms for accelerating the rate of water and energy efficiency retrofits in Australian public buildings: Hybrid bayesian network and system dynamics modelling approach. Appl. Energy 210 , 409–419 (2018).
Toth, E., Bragalli, C. & Neri, M. Assessing the significance of tourism and climate on residential water demand: Panel-data analysis and non-linear modelling of monthly water consumptions. Environ. Modelling Softw. 103 , 52–61 (2018).
Trumbo, C. W. & O’Keefe, G. J. Intention to conserve water: Environmental values, reasoned action, and information effects across time. Soc. Nat. Resour. 18 , 573–585 (2005).
Russell, S. V. & Knoeri, C. Exploring the psychosocial and behavioural determinants of household water conservation and intention. Int. J. Water Resour. Development 36 , 940–955 (2020).
Makki, A. A., Stewart, R. A., Panuwatwanich, K. & Beal, C. Revealing the determinants of shower water end use consumption: Enabling better targeted urban water conservation strategies. J. Cleaner Prod. 60 , 129–146 (2013).
Millock, K. & Nauges, C. Household adoption of water-efficient equipment: The role of socio-economic factors, environmental attitudes and policy. Environ. Resour. Econ. 46 , 539–565 (2010).
Cooper, B., Burton, M. & Crase, L. Urban water restrictions: Attitudes and avoidance. Water Resour. Res. 47 (2011).
Willis, R. M., Stewart, R. A., Panuwatwanich, K., Williams, P. R. & Hollingsworth, A. L. Quantifying the influence of environmental and water conservation attitudes on household end use water consumption. J. Environ. Manag. 92 , 1996–2009 (2011).
Polebitski, A. S. & Palmer, R. N. Analysis and predictive models of single-family customer response to water curtailments during drought 1. JAWRA J. American Water Resour. Assoc. 49 , 40–51 (2013).
Cooper, B. What drives compliance? An application of the theory of planned behaviour to urban water restrictions using structural equation modelling. Appl. Econ. 49 , 1426–1439 (2017).
Cominola, A. et al. Long-term water conservation is fostered by smart meter-based feedback and digital user engagement. npj Clean Water 4 , 1–10 (2021).
Jordán-Cuebas, F. et al. Understanding apartment end-use water consumption in two green residential multistory buildings. J. Water Resour. Plan. Manag. 144 , 04018009 (2018).
Xenochristou, M., Hutton, C., Hofman, J. & Kapelan, Z. Water demand forecasting accuracy and influencing factors at different spatial scales using a gradient boosting machine. Water Resour. Res. 56 , e2019WR026304 (2020).
Corral-Verdugo, V., Frias-Armenta, M., Pérez-Urias, F., Orduña-Cabrera, V. & Espinoza-Gallego, N. Residential water consumption, motivation for conserving water and the continuing tragedy of the commons. Environ. Manag. 30 , 527–535 (2002).
Syme, G. J., Shao, Q., Po, M. & Campbell, E. Predicting and understanding home garden water use. Landsc. Urb. Plan. 68 , 121–128 (2004).
Landon, A. C., Kyle, G. T. & Kaiser, R. A. An augmented norm activation model: The case of residential outdoor water use. Soc. Nat. Resour. 30 , 903–918 (2017).
Domene, E. & Saurí, D. Urbanisation and water consumption: Influencing factors in the metropolitan region of Barcelona. Urb. Stud. 43 , 1605–1623 (2006).
Ashoori, N., Dzombak, D. A. & Small, M. J. Modeling the effects of conservation, demographics, price, and climate on urban water demand in Los Angeles, California. Water Resour. Manag. 30 , 5247–5262 (2016).
Di Mauro, A., Cominola, A., Castelletti, A. & Di Nardo, A. Urban water consumption at multiple spatial and temporal scales. a review of existing datasets. Water 13 , 36 (2021).
Kossieris, P. & Makropoulos, C. Exploring the statistical and distributional properties of residential water demand at fine time scales. Water 10 , 1481 (2018).
Bolorinos, J., Ajami, N. K. & Rajagopal, R. Consumption change detection for urban planning: Monitoring and segmenting water customers during drought. Water Resour. Res. 56 , e2019WR025812 (2020).
Romano, G., Salvati, N. & Guerrini, A. Estimating the determinants of residential water demand in Italy. Water 6 , 2929–2945 (2014).
Adamowski, J. F. Peak daily water demand forecast modeling using artificial neural networks. J. Water Resour. Plan. Manag. 134 , 119–128 (2008).
Marzano, R. et al. Determinants of the price response to residential water tariffs: Meta-analysis and beyond. Environmental Modelling Softw. 101 , 236–248 (2018).
Zounemat-Kermani, M. et al. Neurocomputing in surface water hydrology and hydraulics: A review of two decades retrospective, current status, and future prospects. J. Hydrol. 125085 (2020).
Liu, J., Savenije, H. H. & Xu, J. Forecast of water demand in Weinan city in China using WDF-ANN model. Phys. Chemis. Earth, Parts A/B/C 28 , 219–224 (2003).
Babel, M. S. & Shinde, V. R. Identifying prominent explanatory variables for water demand prediction using artificial neural networks: a case study of Bangkok. Water Resour. Manag. 25 , 1653–1676 (2011).
Quesnel, K. J., Ajami, N. & Marx, A. Shifting landscapes: decoupled urban irrigation and greenness patterns during severe drought. Environmental Res. Lett. 14 , 064012 (2019).
Sarni, W., White, C., Webb, R., Cross, K. & Glotzbach, R. Digital water: Industry leaders chart the transformation journey. Int. Water Assoc. Xylem Inc. https://iwa-network.org/publications/digital-water/ (2019).
Llausàs, A. & Saurí, D. A research synthesis and theoretical model of relationships between factors influencing outdoor domestic water consumption. Soc. Nat. Resour. 30 , 377–392 (2017).
Jorgensen, B. S., Martin, J. F., Pearce, M. W. & Willis, E. M. Predicting household water consumption with individual-level variables. Environ. Behav. 46 , 872–897 (2014).
Makki, A. A., Stewart, R. A., Beal, C. D. & Panuwatwanich, K. Novel bottom-up urban water demand forecasting model: revealing the determinants, drivers and predictors of residential indoor end-use consumption. Resour., Conserv. Recycling 95 , 15–37 (2015).
Mazzoni, F. et al. Investigating the characteristics of residential end uses of water: A worldwide review. Water Res. 230 , 119500 (2023).
Download references
Acknowledgements
The authors acknowledge support by the German Research Foundation and the Open Access Publication Funds of the Technical University of Berlin for covering publication costs.
Open Access funding enabled and organized by Projekt DEAL.
Author information
Authors and affiliations.
Chair of Smart Water Networks, Technische Universität Berlin, Berlin, Germany
A. Cominola
Einstein Center Digital Future, Berlin, Germany
School of Architecture and Civil Engineering, University of Adelaide, Adelaide, SA, Australia
L. Preiss, M. Thyer & H. R. Maier
Victoria University, Melbourne, Australia
School of Engineering and Built Environment, Griffith University, Gold Coast, Australia
R. A. Stewart
Cities Research Institute, Griffith University, Gold Coast, Australia
Department of Electronics, Information, and Bioengineering, Politecnico di Milano, Milan, Italy
A. Castelletti
You can also search for this author in PubMed Google Scholar
Contributions
M.T. and H.M. designed the research. L.P. collected and analyzed most of the papers, and drafted an early-stage outline of this paper. All authors helped collect and review the papers in the final selection. A. C. (Andrea Cominola), L.P., M.T., H.M., P.P., R.S., and A.C. (Andrea Castelletti) developed the review framework. A.C. (Andrea Cominola), L.P., M.T., A.C. (Andrea Castelletti), and H.M. developed the final version of the paper. All authors reviewed the manuscript.
Corresponding authors
Correspondence to A. Cominola or M. Thyer .
Ethics declarations
Competing interests.
The authors declare no competing interests.
Additional information
Publisher’s note Springer Nature remains neutral with regard to jurisdictional claims in published maps and institutional affiliations.
Supplementary information
Supplementary information, rights and permissions.
Open Access This article is licensed under a Creative Commons Attribution 4.0 International License, which permits use, sharing, adaptation, distribution and reproduction in any medium or format, as long as you give appropriate credit to the original author(s) and the source, provide a link to the Creative Commons license, and indicate if changes were made. The images or other third party material in this article are included in the article’s Creative Commons license, unless indicated otherwise in a credit line to the material. If material is not included in the article’s Creative Commons license and your intended use is not permitted by statutory regulation or exceeds the permitted use, you will need to obtain permission directly from the copyright holder. To view a copy of this license, visit http://creativecommons.org/licenses/by/4.0/ .
Reprints and permissions
About this article
Cite this article.
Cominola, A., Preiss, L., Thyer, M. et al. The determinants of household water consumption: A review and assessment framework for research and practice. npj Clean Water 6 , 11 (2023). https://doi.org/10.1038/s41545-022-00208-8
Download citation
Received : 15 September 2021
Accepted : 15 December 2022
Published : 20 February 2023
DOI : https://doi.org/10.1038/s41545-022-00208-8
Share this article
Anyone you share the following link with will be able to read this content:
Sorry, a shareable link is not currently available for this article.
Provided by the Springer Nature SharedIt content-sharing initiative
Quick links
- Explore articles by subject
- Guide to authors
- Editorial policies
Sign up for the Nature Briefing newsletter — what matters in science, free to your inbox daily.

Get the Facts: Data and Research on Water Consumption
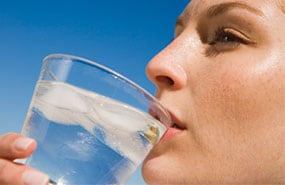
Plain water consumption varies by age, race/ethnicity, and socioeconomic status.
- During 2015–2018, US children and adolescents drank an average of 23 ounces of plain water daily, and US adults drank an average of 44 ounces.
- Among US children and adolescents, plain water intake is significantly lower in younger children, non-Hispanic Black children or Hispanic children (compared to non-Hispanic White children), those living in lower-income households, youth whose head of household had less than a high school education (compared to college or higher), and those who are underweight or normal weight (compared to those with obesity) (Table 1).
- Among US adults, plain water intake is significantly lower in older adults, non-Hispanic Black adults (compared to non-Hispanic White adults), adults with lower income and lower education, and adults without obesity (Table 2).
Top of Page
Mean Daily Plain Water a Intakes Among US Children and Adolescents Aged 2 to 19 Years, National Health and Nutrition Examination Survey 2015–2018 b
Mean daily plain water a intakes among us adults aged 20 years and older, national health and nutrition examination survey 2015–2018 b.
- Increasing Access to Drinking Water and Other Healthier Beverages in Early Care and Education Settings [PDF-3.68MB]
- Increasing Access to Drinking Water in Schools
- Water Access in Schools Microlearning Modules guide schools to help students drink more water during the day.
- Nutrition and Obesity Policy Research and Evaluation Network (NOPREN) Water Access Working Group : This site provides information on this working group who focus on policies and economic issues regarding free and safe drinking water access.
- Environmental Protection Agency (EPA)— Lead in Drinking Water at Schools and Childcare Facilities : This site provides information about lead in drinking water at schools and child care facilities.
- Environmental Protection Agency (EPA) – Find Your Local Consumer Confidence Report : This site provides information on annual drinking water quality reports from local water suppliers.
- Private Drinking Water Wells |US EPA :
This site provides information for private well owners. Private well owners are responsible for the safety of their water. This website educates well owners on wells, groundwater, and information on protecting their health. It is estimated that more than 13 million households rely on private wells for drinking water in the United States (US Census American Housing Survey 2017).
- Associations between household water fluoridation status and plain tap or bottled water consumption; September 2020
- Correlates of infrequent plain water intake among US high school students: National Youth Risk Behavior Survey, 2017; March 2020
- Dietary and physical activity behaviors among high school students – Youth Risk Behavior Survey, United States, 2019; August 2020
- A water availability intervention in New York City public schools: influence on youths’ water and milk behaviors; February 2015
- Student-reported school drinking fountain availability by youth characteristics and state plumbing codes; April 2014
- Perceptions of tap water and school water fountains and association with intake of plain water and sugar-sweetened beverages; March 2014
- Factors associated with low water intake among U.S. high school students – National Youth Physical Activity and Nutrition Study, 2010; September 2012
- Factors related to water filter use for drinking tap water at home and its association with consuming plain water and sugar-sweetened beverages among US adults; Jan 2022
- Perceptions of drinking water safety and their associations with plain water intake among U.S. Hispanic adults; August 2019
- Community-based policies and support for free drinking water access in outdoor areas and building standards in U.S. municipalities; April 2018
- The relationship of perceptions of tap water safety with intake of sugar-sweetened beverages and plain water among U.S. adults; January 2014
- Behaviors and attitudes associated with low drinking water intake among U.S. adults, Food Attitudes and Behaviors Survey, 2007; April 2013
To receive email updates about this topic, enter your email address.
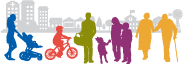
- Physical Activity
- Overweight & Obesity
- Healthy Weight, Nutrition, and Physical Activity
- Breastfeeding
- Micronutrient Malnutrition
- State and Local Programs
- Food Safety
- Healthy Schools – Promoting Healthy Behaviors
- BAM! Body and Mind Resources for Teachers
Exit Notification / Disclaimer Policy
- The Centers for Disease Control and Prevention (CDC) cannot attest to the accuracy of a non-federal website.
- Linking to a non-federal website does not constitute an endorsement by CDC or any of its employees of the sponsors or the information and products presented on the website.
- You will be subject to the destination website's privacy policy when you follow the link.
- CDC is not responsible for Section 508 compliance (accessibility) on other federal or private website.
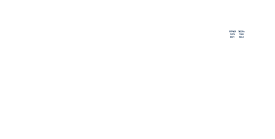
Water Use and Stress
How much water do we use? How did it change over time?
By Hannah Ritchie and Max Roser
First published in 2015; most recent substantial revision in February 2024.
This article previously covered aspects of clean water and sanitation access; you can now find this material in our topic pages on Clean Water and Sanitation .
Other research and writing on water use and stress on Our World in Data:
- Dairy vs. plant-based milk: what are the environmental impacts?
- What are the environmental impacts of food and agriculture?
Freshwater use
Global freshwater use.
A growing global population and economic shift towards more resource-intensive consumption patterns means that global freshwater use has increased approximately six-fold since 1900. Freshwater use includes freshwater withdrawals for agriculture, industry, and municipal uses. This is shown in the chart. Rates of global freshwater use increased sharply from the 1950s onwards but since 2000 appear to be plateauing, or at least slowing.
Freshwater withdrawals by country
This breakdown of total freshwater withdrawals is shown by country in the chart. In recent years, India, China, and the United States are the three countries with the largest freshwater withdrawals.
Water withdrawals per capita
Levels of water use vary significantly across the world. The visualization shows the average level of water withdrawal per person per year. As described in detail in our Data Quality & Definitions section , water withdrawal is defined as the quantity of freshwater taken from groundwater or surface water sources (such as lakes or rivers) for use in agricultural, industrial, or domestic purposes.
As shown, there is a large variance in levels of water withdrawal rates across the world - this can depend on a range of factors, including climate and the importance of a country's agricultural or industrial sector, as explored in the sections below.
Renewable freshwater resources
Renewable freshwater resources per capita.
To maintain sustainable levels of water resources, rates of water withdrawals must be below rates of freshwater replenishment. 'Renewable internal freshwater flows' refer to internal renewable resources (internal river flows and groundwater from rainfall) in a country.
Renewable internal flows are, therefore, an important indicator of water security or scarcity. If rates of freshwater withdrawal begin to exceed the renewable flows, resources begin to decline. The chart shows the level of renewable internal freshwater resources per capita.
Per capita renewable resources depend on two factors: the total quantity of renewable flows and the size of the population. If renewable resources decline — as can happen frequently in countries with large annual variability in rainfall, such as monsoon seasons — then per capita renewable withdrawals will also fall. Similarly, if total renewable sources remain constant, per capita levels can fall if a country's population grows.
As we see, per capita renewable resources are declining in many countries, mainly as a result of population increases.
Per capita renewable water resources by region
The chart shows the average per capita renewable freshwater resources across geographical regions, measured in cubic meters per person per year.
Quantity of renewable freshwater resources by region
The chart shows the total internal renewable freshwater resources by region.
Agricultural water withdrawals
Freshwater use in agriculture.
Water is an essential input to global agriculture, whether in the form of rainfed sources or pumped irrigation. The visualization shows the total quantity of freshwater withdrawals that are used in agriculture, whether in the form of food crops, livestock, biofuels, or other non-food crop production.
India's agricultural water consumption has been growing rapidly — almost doubling between 1975 and 2010 — as its population and total food demand continue to increase.
Share of freshwater withdrawals used in agriculture
How do agricultural freshwater withdrawals compare to industrial and domestic sources? Globally, we use approximately 70 percent of freshwater withdrawals for agriculture. 1
However, this share varies significantly by country – as shown in the chart, which measures the percentage of total freshwater withdrawals used for agriculture. Here, we see large variations geographically and by income level. The average agricultural water use for low-income countries is much higher than it is for high-income countries.
There are a number of countries across South Asia, Africa, and Latin America that use more than 80 percent of water withdrawals for agriculture. Many countries in Europe tend to use a much lower share of water for agriculture; Germany and the Netherlands use less than one percent.
What share of agricultural land is irrigated?
Irrigation — the deliberate supply of agricultural land with water — has been an important input factor in the observed increase of crop yields across many countries in recent decades. It has also been a strong driver in the quantity of water used for agriculture.
The share of the total agricultural area (the combination of arable and grazing land) that is irrigated is shown in the chart. As we can see, irrigation is particularly prevalent across South Asia and the Middle East, with many countries irrigating more than a fifth of their agricultural area.
Slower progress in increasing crop yields in recent decades in Sub-Saharan Africa has been partly attributed (among other factors including fertilizer application rates and crop varieties) to lower uptake of irrigation in Sub-Saharan Africa. 2
Industrial water withdrawals
Water use in industry.
Water is used for a range of industrial applications , including dilution, steam generation, washing, and cooling of manufacturing equipment. Industrial water is also used as cooling water for energy generation in fossil fuel and nuclear power plants (hydropower generation is not included in this category) or as wastewater from certain industrial processes.
The visualization shows the total annual water withdrawals which are used for industrial purposes. The United States and China are two countries that withdraw the most water for industrial uses.
Many countries across the Americas, Europe, and East Asia & Pacific regions use more than one billion m³ for industrial uses per year. Freshwater withdrawal for industrial uses is typically much lower across Sub-Saharan Africa.
Share of freshwater withdrawals used in industry
The visualization provides an overview of industrial water withdrawals measured as the share of total water withdrawals (which is the sum of agricultural, industrial, and domestic uses).
In contrast to the global distribution of agricultural water withdrawals, industrial water usage tends to dominate in high-income countries and is small in low-income countries.
The regions with the greatest share are Central and Eastern Europe. Across Sub-Saharan Africa, these industrial water withdrawals contribute to a very small share of total withdrawals.
Municipal water withdrawals
Freshwater use for households and public services.
Municipal water is defined as the water we use for domestic, household purposes, or public services. This is typically the most 'visible' form of water: the water we use for drinking, cleaning, washing, and cooking.
Municipal water withdrawals are shown in the chart. China, India, and the United States have the highest domestic water demands. This is partly down to the large populations of these countries and higher per capita water demands in the United States.
Share of freshwater withdrawals used in households
Despite being the most visible use of freshwater, domestic demands for most countries are small relative to agricultural and industrial applications. Globally, around 10 percent of withdrawals are used for municipal purposes.
Municipal uses as a share of total water withdrawals across the world are shown in the chart. The majority of countries use less than 30 percent of withdrawals for domestic purposes.
The share of municipal water in some countries across Sub-Saharan Africa can be high as a result of very low demands for agricultural and industrial withdrawals. Domestic uses of water withdrawals can also dominate in some countries across Europe with high rainfall, such as the United Kingdom and Ireland, where agricultural production is often largely rainfed and industrial output is low.
Water stress and scarcity
As the global population grows (increasing agricultural, industrial, and domestic demands for water) and water demand increases, water stress and the risk of water scarcity are now a common concern. This is even more applicable for particular regions with lower water resources and/or larger population pressures.
Water stress is defined based on the ratio of freshwater withdrawals to renewable freshwater resources. Water stress does not insinuate that a country has water shortages, but it does give an indication of how close it may be to exceeding a water basin's renewable resources. If water withdrawals exceed available resources (i.e., greater than 100 percent), then a country is either extracting beyond the rate at which aquifers can be replenished or has very high levels of desalinization water generation (the conversion of seawater to freshwater using osmosis processes).
What share of freshwater resources do we use?
The visualization provides a measure of levels of water stress across the world. This is measured based on freshwater withdrawals as a share of internal (renewable) resources. The Food and Agriculture Organization of the United Nations categorizes water stress in the following ways: if withdrawals are less than 25 percent of resources, then a country has no water stress; 25-50 percent is low stress; 50-75 percent is medium; 75-100 percent high stress; and greater than 100 percent is critical stress. 3
Several countries across the Middle East & North Africa have extremely high levels of water stress. Many have withdrawal rates well in excess of 100 percent — this means they are either extracting unsustainably from existing aquifer sources or producing a large share of water from desalinization.
Water stress in Northern Europe, Canada, much of Latin America, Sub-Saharan Africa, and Oceania is typically low or low to medium.
Water use of food products
Globally, 70 percent of freshwater withdrawals are used for agriculture. However, water requirements vary significantly depending on food type. The charts show the global average freshwater withdrawals in liters per kilogram of food product, per 1000 kilocalories, and 100 grams of protein.
Freshwater withdrawals per kilogram of food product
Freshwater withdrawals per 1000 kilocalories, freshwater withdrawals per 100 grams of protein, definitions, water withdrawals.
Water withdrawal: Water withdrawals (also sometimes known as 'water abstractions') are defined as freshwater taken from ground or surface water sources (such as rivers or lakes), either permanently or temporarily, and used for agricultural, industrial, or municipal (domestic) uses.
The UN Food and Agricultural Organization (FAO) AQUASTAT Database defines total water withdrawal as: "Annual quantity of water withdrawn for agricultural, industrial and municipal purposes. It can include water from primary renewable and secondary freshwater resources, as well as water from over-abstraction of renewable groundwater or withdrawal from fossil groundwater, direct use of agricultural drainage water, direct use of (treated) wastewater, and desalinated water. It does not include in-stream uses, which are characterized by a very low net consumption rate, such as recreation, navigation, hydropower, inland capture fisheries, etc."
Total withdrawal is equal to: [withdrawals for agriculture] + [withdrawals for industry] + [withdrawals for municipal/domestic uses].
The UN Food and Agricultural Organization (FAO) AQUASTAT Database gives the following definitions for agricultural, industrial, and municipal withdrawals:
Agricultural water withdrawal : "Annual quantity of self-supplied water withdrawn for irrigation, livestock and aquaculture purposes. It can include water from primary renewable and secondary freshwater resources, as well as water from over-abstraction of renewable groundwater or withdrawal from fossil groundwater, direct use of agricultural drainage water, direct use of (treated) wastewater, and desalinated water. Water for the dairy and meat industries and industrial processing of harvested agricultural products is included under industrial water withdrawal."
Industrial water withdrawal : "Annual quantity of self-supplied water withdrawn for industrial uses. It can include water from primary renewable and secondary freshwater resources, as well as water from over-abstraction of renewable groundwater or withdrawal from fossil groundwater, direct use of agricultural drainage water, direct use of (treated) wastewater, and desalinated water. This sector refers to self-supplied industries not connected to the public distribution network. The ratio between net consumption and withdrawal is estimated at less than 5%. It includes water for the cooling of thermoelectric and nuclear power plants, but it does not include hydropower. Water withdrawn by industries that are connected to the public supply network is generally included in municipal water withdrawal."
Municipal water withdrawal : "Annual quantity of water withdrawn primarily for the direct use by the population. It can include water from primary renewable and secondary freshwater resources, as well as water from over-abstraction of renewable groundwater or withdrawal from fossil groundwater, direct use of agricultural drainage water, direct use of (treated) wastewater, and desalinated water. It is usually computed as the total water withdrawn by the public distribution network. It can include part of the industries and urban agriculture, which is connected to the municipal network. The ratio between the net consumption and the water withdrawn can vary from 5 to 15% in urban areas and 10 to 50% in rural areas."
Water stress levels
Renewable internal freshwater resources refer to the quantity of internal freshwater from inflowing river basins and recharging groundwater aquifers. Data on renewable resources should be treated with caution; since this data is gathered intermittently, it fails to capture seasonal and annual variance in water resources, which can be significant in some nations. Data at a national level also fails to capture variability at more local levels, which can be important when analyzing the sustainability of particular groundwater aquifers or surface water basins.
Water stress is defined in its simplest terms as occurring when total freshwater withdrawal substantiates a large share of available renewable freshwater resources.
UN-Water defines water stress categories based on this percentage (% of withdrawals to renewable resources) as follows:
- <25% = no stress
- 25-50% = low stress
- 50-75% = medium stress
- 75-100% = high stress
- >100% = critical stress
Interactive Charts on Water Use and Stress
Gleick,P.H et al. (2014). The World's Water: The Biennial Report on Freshwater Resources. Washington, DC: Island Press). Available online .
World Bank (2008) – World Development Report (2008): Agriculture for Development. Washington, DC: World Bank. Available online .
FAO and UN Water. 2021. Progress on Level of Water Stress. Global status and acceleration needs for SDG
Indicator 6.4.2, 2021. Rome. https://doi.org/10.4060/cb6241en
Cite this work
Our articles and data visualizations rely on work from many different people and organizations. When citing this topic page, please also cite the underlying data sources. This topic page can be cited as:
BibTeX citation
Reuse this work freely
All visualizations, data, and code produced by Our World in Data are completely open access under the Creative Commons BY license . You have the permission to use, distribute, and reproduce these in any medium, provided the source and authors are credited.
The data produced by third parties and made available by Our World in Data is subject to the license terms from the original third-party authors. We will always indicate the original source of the data in our documentation, so you should always check the license of any such third-party data before use and redistribution.
All of our charts can be embedded in any site.
Our World in Data is free and accessible for everyone.
Help us do this work by making a donation.
- Previous Article
- Next Article
INTRODUCTION
Results and discussion, conclusions, acknowledgements, data availability statement, defining domestic water consumption based on personal water use activities.

- Article contents
- Figures & tables
- Supplementary Data
- Open the PDF for in another window
- Guest Access
- Cite Icon Cite
- Permissions
- Search Site
Melissa Lauren Crouch , Heinz Erasmus Jacobs , Vanessa L. Speight; Defining domestic water consumption based on personal water use activities. AQUA - Water Infrastructure, Ecosystems and Society 1 November 2021; 70 (7): 1002–1011. doi: https://doi.org/10.2166/aqua.2021.056
Download citation file:
- Ris (Zotero)
- Reference Manager
As the world increasingly faces water shortages, it is critical to develop realistic estimates of water consumption based on water-use activities rather than top-down imposed limits. This study quantifies the absolute basic consumption (ABC) of an individual, irrespective of water-use location or water source, with a focus on personal water-use activities rather than measured household consumption. A theoretical model to stochastically describe 21 personal water-use activities was developed, corresponding to lifestyle levels based upon Maslow's hierarchy of physiological needs. From this model, the expected value of ABC was 92 L/capita/day (LPCD) and includes restrictions on several everyday activities and thus would be better as a minimum target during stringent water restrictions. A more realistic expected value for non-wasteful, indoor-only water use was determined to be 175 LPCD. The results of this study serve as a useful benchmark to inform policy choices and efforts to conserve water without affecting health and wellbeing.
It is important to quantify water consumption based on water-use activities and across all daily water-use locations.
The expected value of basic water consumption for a healthy urban lifestyle is 92 L/person/day, which includes restrictions on everyday activities.
A more realistic expected value for water consumption is 175 L/person/day across all water-use locations during a typical day.
Graphical Abstract

Resource management, particularly water, is a key challenge to urban sustainability. The supply of adequate water is essential to ensure healthy urban living, but access to clean water still evades millions of people around the globe ( WHO & UNICEF 2021 ). At a basic level, water is required for direct consumption, food preparation as well as for hygiene purposes. A number of recent drought emergencies around the world, notably the City of Cape Town's ‘Day Zero’ crisis, have brought greater attention to the need to reduce water use and quantify the minimum water needs for urban residents ( Booysen et al. 2019 ). In contrast, the COVID-19 world health crisis of 2020–2021 has placed emphasis on hygiene in general – also regular and proper hand washing and cleaning of surfaces – in order to prevent or minimise the spread of infectious diseases. Even absent a crisis, many jurisdictions are moving to establish targets for maximum domestic water consumption (e.g. Defra 2019 ), but these targets can often represent arbitrary ideal values.
While it is critical to understand and limit water wastage, the current approaches to addressing household water consumption focus almost exclusively on driving down demand, with a focus on water-use appliances and fixtures – without considering human needs and the personal water-use activities that these products enable. It is these water-use activities that deliver a variety of benefits for public health (e.g. hand washing, sanitation, cleanliness) and wellbeing (e.g. gardening, pet care, relaxation, physical exercise) that are associated with an elevated standard of modern urban living.
Context and limitations
This paper presents a method for exploring water requirements in the context of modern living standards as a benchmark for holistically understanding water needs, given current technology, so that efforts to promote water efficiency are realistic and do not adversely impact health and wellbeing. Individual domestic water use was described in this study as a function of specific water-use activities, each linked to stochastic input parameters. Other variables that are known to affect household water use, such as price (e.g., Howe & Linaweaver 1967 ), income (e.g., DHS 2019 ), plot area (e.g., Makwiza & Jacobs 2016 ), and rainfall (e.g., Linaweaver et al. 1963 ), were purposefully excluded. The focus in this study was on the individual's personal water-use activities in any setting (at home, the office, a restaurant, the gymnasium, etc.) instead of water use at a fixed location and cost, i.e. at the particular home. Also, the impact of the different consumption levels on the water service provider, for example on the utility revenue due to reduced water sales, was considered beyond the scope of this study.
Minimum water requirements
Humans have the right to ‘sufficient, safe, acceptable, physically accessible and affordable water for personal and domestic uses’ ( United Nations Committee on Economic, Social and Cultural Rights 2003 ). A minimum quantity of water should be available for drinking, food preparation, basic hygiene, bathing and sanitation to ensure sustainable, healthy urban living. The most basic water requirement is stipulated by the World Health Organization (WHO) and varies between 20 and 50 litres per capita per day (LPCD). The basic water requirement determined by WHO is limited to access at an offsite tap and does not include consideration of homes with piped water ( WHO 2003 ). For this study, water use is viewed from the perspective of sustainable, healthy urban living which implies a higher level of service than the minimum required to survive, that is a full household connection to continuously supplied, piped, pressurised, potable water. Water quality considerations were beyond the scope of this study.
Water use and human needs
Willis et al. (2011) classified types of household water uses as being non-discretionary or discretionary, although this distinction is subjective and context-specific. Under this definition, non-discretionary water use is 40–70 LPCD and any water use above this value should be considered discretionary water use, irrespective of what it is being used for. A number of studies have examined household water use, using metering data, surveys and other methods, yielding typical values from 93 LPCD ( Athuraliya et al. 2012 ) to 430 LPCD ( Hay et al. 2012 ). Biswas & Gangwar (2020) reported that water supply in Delhi, India, varies from 29 LPCD in regions with poor service to 509 LPCD in the privileged residential zones. A key determinant in these reported values is the extent of outdoor water use, which can vary significantly based upon the local climate and building preferences, making it difficult to compare values from different areas and different studies. Also, water demand management and conservation efforts could lead to more efficient use of water ( Adler 2011 ), with related water reduction. However, such efficiency improvements are limited by aspects such as current practices, behaviour, available technology and affordability. Reducing water consumption via demand management practices without impacting the level of service provided to consumers and without negatively affecting healthy, sustainable urban living is a genuine challenge for sustainable cities. In view of the above, a framework is needed to link water use to the expectations of end-users.
Maslow's hierarchy of human needs is a useful framework in which to consider water-use requirements. Humans have different levels of needs, defined by Maslow in a hierarchy ranging from basic physiological needs, safety, love and belonging, and esteem to the highest level of self-actualisation, which is defined as achieving one's full potential ( Maslow 1943 ). Basic needs for water consumption to fulfil baseline physiological and safety needs would include drinking water, cooking uses, basic hygiene, and sanitation. Higher level needs for esteem and self-fulfilment would include additional water uses associated with sparkling clean homes and belongings, water-related sports or relaxation activities requiring swimming pools and hot tubs and irrigated gardens. Clayton (2007) found that gardening has important positive effects on individuals, as well as on the urban ecosystem. Outdoor use can support seasonal small-scale horticultural crops in backyards ( Makwiza & Jacobs 2016 ) and improve general wellbeing, which contribute to overall quality of life.
Water use is intricately linked to health and hygiene ( Prüss-Üstün et al. 2008 ; WHO 2019 ), with insufficient water services leading to diseases such as diarrhoea or other faecal-orally transmitted diseases, skin and eye infections ( Bradley 1977 ). Hand washing, particularly with increased frequency and duration as was recommended to reduce the spread of coronavirus, is a key element of hygiene that uses clean water. Many household activities require hand washing to maintain hygiene and avoid the spread of contamination including contact with an infected person, handling food, coughing or sneezing, making use of the toilet, handling laundry items, handling domestic animals, disposing of household refuse, or caring for an infant. Furthermore, it is essential to regularly clean clothes and household linen as well as surfaces, floors, and household furniture to maintain optimal hygiene ( RSPH 2019 ).
Several countries have had water efficiency legislation in place for decades, although this typically focuses on water efficiency labelling requirements for specific water-use products such as toilets and appliances ( AS/NZS 2016 ; USEPA 2020 ). A few examples are presented here, but this list is not comprehensive. UK Building Regulations currently require that all new homes are built to a water consumption standard of 125 LPCD, with an optional standard of 110 LPCD which can be applied by local authorities in water-stressed areas and has been adopted by approximately 100 areas across the UK ( HM Government 2016 ). Biswas & Gangwar (2020) report on norms and benchmarks prepared by various public institutions in India and report a requirement of 227 LPCD. South African guidelines allow for supply of 25 LPCD for communal standpipes to 400 LPCD for high-income residential areas ( DHS 2019 ).
Like the water efficiency labelling programmes, many building regulation calculators focus on the water-use products and assign a factor for daily usage to each one and, therefore, is best suited for the design of new buildings rather than realistic representation of actual water consumption. The UK Building Regulations ( HM Government 2016 ) acknowledge that human behaviour will influence actual water consumption and as such, these guidelines should not be used for water supply calculations.
Water loss and leakage occur in residential homes from time to time ( Lugoma et al. 2012 ; Meyer et al. 2018 ), where a number of water fixtures and plumbing can leak and often remain undetected. It is often unclear whether allowance for water loss and leakage is included in LPCD values as presented in the published guidelines. Water losses may also be attributed to recommended practices in households such as flushing of lead pipes before drinking the water, which can equate to a loss of 5–20 L per event ( Speight 2018 ), and to wasted water while users wait for a suitable temperature from hot water taps, which has been estimated to be as high as 15 LPCD ( Nawaz & Waya 2014 ). Every home and lifestyle level is subject to a certain degree of water loss and leakage, as are the piped distribution networks which deliver water to households. Ageing infrastructure is a problem in many countries around the world and will exacerbate leakage. In many cases, the network leakage represents a volume similar to an individual's domestic water use. For example, in the UK an estimated 3.1 billion litres per day of water is lost through leakage, which represents 121 L per household per day when averaged across the 26.2 million households served ( Water UK 2019 ). Therefore, efforts to promote water efficiency need to take a balanced approach that considers the impact on health and wellbeing of consumers within the wider context of the water utility's supply and leakage situation; this study addresses this need by developing a stochastic model for individual water use across typical urban water-use activities.
A stochastic model comprising 21 water-use activities described by 135 model input parameters was developed in this study to model per capita personal water use, named the LCD model. The model input parameters drew upon the published literature for household water use and a survey which covered 11 countries worldwide. A comprehensive description of the development of the model is given in Crouch (2020) .
The modelling methodology identified water-use activities and their associated water-use requirements, linked these water-use activities to lifestyle levels, and developed probability distributions for the occurrence of each water-use activity. This study quantifies the water use of an individual, irrespective of water-use location or water source, with a focus on personal water-use activities rather than measured household consumption. Monte Carlo simulations of 100,000 households were performed using the @Risk software in MS Excel for each lifestyle level to determine the expected value of individual water use under the given conditions.
Description of water-use activities
A water-use activity was defined in this study as the specific activity, either indoor or outdoor, for which water is used. Showering, washing clothes, cleaning the house, and car washing are examples of water-use activities. The supply of the water does not have to originate at the point of the activity. For example, a bucket of water can be drawn from the kitchen, shower, or bath tap and used for washing the floors of the house. The water-use activity, in this case, would be cleaning the house.
A water-use activity differs from the commonly encountered water end-use. An end-use is the appliance or plumbing fixture that serves as the water exit point (e.g. tap), regardless of the activity for which the water is being used. Following the previous example, the end-use would be the kitchen, shower, or bath tap, whereas the water-use activity would be cleaning the house. Some water-use activities are intrinsically associated with appliances or fixtures, including dishwashing using a dishwasher, laundry using a washing machine, shower, and toilet.
Domestic water use is affected by a number of factors, including the age, occupation, cultural preferences, and household income of the individuals using water. For example, the age of the occupants affects water use as the activities of an individual change with age and their associated lifestyle ( Browne et al. 2014 ). When showers are used, children and teens have been found to shower for longer than adults, thus increasing household water use ( Mayer et al. 1999 ). In Germany, household water use has also been found to increase with age, often because the elderly who are retired spend more time in the house, which implies an increased likelihood to use water while at home ( Schleich & Hillenbrand 2009 ). For this study, such factors were not explicitly modelled, because water use in this study was not linked to spatial attributes – relevant factors were instead considered in the development of the appropriate stochastic representation of water-use activities.
Based upon the literature from a number of studies on individual water-use activities, household water use, and water end-use, the frequency and volume associated with each of the 21 water-use activities were estimated along with the penetration of the technology. Full details are provided in Supplementary Table S1 and Figures S1–S7.
Lifestyle levels
Based upon Maslow's hierarchy of human needs, five lifestyle levels for water consumption were developed. The first is the minimum water use for basic survival, as defined by the WHO (2003) and similar studies. Typically, the minimum water use does not apply to piped water systems and excludes any outdoor water use.
The second lifestyle level, corresponding to Maslow's second level which includes safety as well as basic physiological wellbeing, is defined as the absolute basic consumption (ABC). The ABC can be considered in this study as the minimum personal daily indoor water requirement for a healthy modern urban lifestyle and was so designated to represent a scenario of water restrictions during a drought or living in a water scarce climate. Outdoor water use, including garden irrigation, swimming pool maintenance, car washing, and cleaning of sports equipment, was not deemed a necessity when considering the minimum water requirements for the ABC lifestyle level.
The realistic everyday allowable level (REAL) consumption was defined to include additional water use to support a more comfortable modern lifestyle than would be the case under ABC, but still excluding outdoor water use. Leakage and water loss were excluded from these lifestyle levels to focus exclusively on the calculation of indoor water use. In cases where leakage is known to be significant, it should be added separately to the ABC and REAL consumption values.
The highest two levels are esteem, which expands upon the uses allowed for REAL consumption and permits some outdoor water use and accounts for leakage, and ultimate, which is unrestricted water use. Leakage and water losses do regularly occur in pipes, toilets, taps, geysers, and swimming pools, at some stage and to some degree, in all households. While leakage and water losses can account for 10–20% of household water use, only 17% of households have significant leakages ( Heinrich 2009 ; Water Corporation 2010 ), so the leakage impacts were restricted to esteem and ultimate lifestyle levels.
A summary description of the water uses associated with lifestyle levels ABC through ultimate is given in Table 1 , with full details of the water-use activities for the REAL level provided in Supplementary Table S1.
Summary of water-use activities included in each lifestyle level
a Restrictions were applied, see Table 2 .
Restrictions for ABC versus REAL consumption
The development of the ABC and REAL lifestyle levels depicts a relatively conservative water use (REAL) and the absolute minimum water use (ABC). The REAL lifestyle level was set up to allow for indoor water use with minimal restrictions, but with no outdoor water use permitted. However, to achieve the ABC lifestyle level within an urban household, serious lifestyle changes would be required such as eliminating indoor plants and shaving. A number of water-use appliances were assumed to be at high efficiency for the ABC lifestyle level, which in reality would require retrofitting and a cost implication. A comparison of the affected water-use activities for the REAL and the ABC lifestyle levels is given in Table 2 .
Restrictions on water-use activities for REAL and ABC lifestyle levels
Household size
Household size varies notably and contributes significantly to domestic water use, having been found to be the most significant factor affecting total household water use ( Rathnayaka et al. 2014 ). The per capita water use of a household decreases as the household size increases, because many water-use activities benefit multiple household members, such as washing machines, cleaning, dishwashers, and cooking.
The model results for a single-person household yield an expected value for REAL consumption of 175 LPCD, with a normal range of 100–251 LPCD ( Table 3 ). The ABC represents a 47% decrease in water use from the REAL lifestyle level, with an expected normal range for ABC of 52–132 LPCD, defined as the mean plus or minus one standard deviation. The stochastic nature of this model means that there is overlap between the REAL and ABC lifestyle levels, which depend on the specific water-use activities that were included in each simulation. These results indicate that in dire situations, placing severe restrictions on water use could result in a reduction in water use of up to 47% from normal indoor water use. However, there would be considerable cost and lifestyle habit implications, including retrofit of appliances and limited water use for various activities. In view of additional hygiene requirements that would be linked to a pandemic (e.g. COVID-19), the ABC would be unachievable.
Predicted water use for a single-person household at each lifestyle level
The results ( Table 3 ) were compared to the literature values reported from over 100 international studies for per capita water use ranked from lowest to highest, as shown in Figure 1 . The mean ABC is consistent with the lowest reported per capita water use, which reinforces the restricted nature of the requirements to achieve the ABC level. The ultimate consumption lifestyle-level expected value is lower than several studies. The predicted normal use range for the ultimate level encompasses all but the single highest reported per capita water use. The LCD model delivers a realistic and representative prediction of individual water use when compared with numerous previous studies.

Comparison of predicted water use to literature values for per capita water use.
The results were adjusted for an increase in household size by using Equation ( 1 ), with maximum household size being limited to five people per household in this analysis. The results of the expected per capita consumption with increase in household size, for each lifestyle level, are given in Figure 2 . The per capita water use decreases with an increasing number of household members, and the biggest difference observed is for the ultimate lifestyle level where large water uses such as outdoor irrigation are mitigated by providing benefit to a larger number of people. The ABC lifestyle level is able to meet a target of 50 LPCD, which was the goal during the Cape Town ‘Day Zero’ extreme drought, when the household size is increased to five people.

Predicted water use adjusted for household size.
The results of this study demonstrate that one-time initiatives providing incentives to retrofit old toilets, shower heads, and faucets may reduce the average indoor water use, but to remain at the ABC level of 92 LPCD, lifestyle changes are also necessary. The lifestyle changes selected for this analysis include: only flushing the toilet twice per day, never shaving with water, using the beaker technique to brush teeth, using hand sanitiser to wash hands when they are not soiled, and limiting showers to 3 min regardless of water temperature. Many of these practices have been included in drought management plans around the world and are tolerated by water users in the short term but would not be considered desirable on a permanent basis. For the current developed world lifestyle and typical household water-use fixtures, the REAL lifestyle level of water consumption of 175 LPCD is more realistic – in cases where outdoor use could be completely banned.
Consideration should also be made for location. The ABC and REAL lifestyle levels do not account for any outdoor water use, including water to wash cars. For households with no outdoor spaces and/or cars, achieving REAL consumption levels is realistic. However, in countries where outdoor green spaces require irrigation, completely restricting outdoor water use to achieve reduced water consumption may prove socially unacceptable, or even impossible in the long term. Many water scarce parts of the world already restrict outdoor usage to certain days to help manage the impact of this potentially large area of water consumption. But as urban gardening grows in popularity, especially for food production, and climate change impacts necessitate more irrigation ( Makwiza et al. 2018 ), outdoor urban water use will become more contentious, particularly if potable water sources are to be used for this purpose.
The approach taken in this study to focus on water-use activities rather than measured household water consumption makes an important advance into understanding realistic ways to conserve water. However, some variables known to affect demand were thus not considered in this study (e.g., water price and garden size). The LCD model with the four lifestyle levels of consumption that were defined in this study illustrate a range of possible choices in terms of restrictions for different water-use activities, but other options could be modelled to evaluate policy choices and their impact on individual water consumption.
The quantification of ABC for healthy urban living was based on current technology, ignoring potential future efficiency improvements and leakage. The resulting ABC of 92 LPCD for a single-person household places a number of lifestyle restrictions on consumers. The long-term sustainability of consumption at such a level using current technology is a concern and hygiene would be negatively impacted through reduced toilet flushing and hand washing. Thus, it is more appropriate that the ABC consumption level be considered as a short-term solution in times of severe water scarcity or until efficiency improvements become available. A more feasible lifestyle level for urban water users with indoor water use only is the REAL consumption, with an expected value of 175 LPCD for a single-person household. Many European countries have already achieved an average per capita water consumption lower than this value without compromising a healthy urban lifestyle, although national consumption values reflect a majority of multi-person households. For a two-person or three-person household, the REAL consumption equates to 129 and 108 LPCD, respectively. A national target for water efficiency within this range seems realistic for areas where outdoor water use could be eliminated.
There is a need to take a holistic view of water use, including leakage and other inefficiencies, to meet future challenges with regard to water scarcity and healthy urban living. A focus solely on household consumption, often as quantified through consumer meter readings, fails to capture the wide range of water-use activities that an individual performs away from the household. An important consideration for policy-makers is that a strong emphasis on reduction of household consumption can increase water consumption away from the home, e.g., in the office, shopping centres, sports facilities, and gyms. The offsite use of water for household activities could lead to increased water use per person, because the person using the water does not pay for consumption away from home. Anecdotally, during the Cape Town drought as increased scrutiny was placed on metered household water consumption, people began to shower at work or the gym to avoid the appearance of high water consumption and related fines at home (the authors had first-hand experience of this). The issue of water consumption at non-domestic locations also reinforces the need to consider water conservation at commercial and industrial premises, where measures such as water recycling at commercial car washes can deliver water savings to a large number of individuals and preserve potable sources for water-use activities that require the cleanest water.
The ABC level of consumption could imply lifestyle changes that are not feasible, given current practices, available technology, affordability, and the levels of service expected by urban consumers in many developed countries. A more feasible lifestyle level for urban water users with indoor water use only is the REAL consumption, with an expected value of 175 LPCD for a single-person household. This study was not done with the assumption that the lifestyle levels or specific water-use activities could be dictated, but rather to inform the kinds of lifestyle changes that may be required in relation to future water-use targets issued by authorities.
This research was funded by the UK Engineering and Physical Sciences Research Council under grant EP/N010124/1 and by the South African Water Research Commission under the WRC special call, grant K5/29/80/13.
All relevant data are included in the paper or its Supplementary Information.
Supplementary data
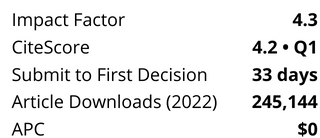
Affiliations
- ISSN 2709-8028 EISSN 2709-8036
- Open Access
- Collections
- Subscriptions
- Subscribe to Open
- Editorial Services
- Rights and Permissions
- Sign Up for Our Mailing List
- IWA Publishing
- Republic – Export Building, Units 1.04 & 1.05
- 1 Clove Crescent
- London, E14 2BA, UK
- Telephone: +44 208 054 8208
- Fax: +44 207 654 5555
- IWAPublishing.com
- IWA-network.org
- IWA-connect.org
- Cookie Policy
- Terms & Conditions
- Get Adobe Acrobat Reader
- ©Copyright 2021 IWA Publishing
This Feature Is Available To Subscribers Only
Sign In or Create an Account
- Review Paper
- Open access
- Published: 28 April 2021
The relationship between plant growth and water consumption: a history from the classical four elements to modern stable isotopes
- Oliver Brendel ORCID: orcid.org/0000-0003-3252-0273 1
Annals of Forest Science volume 78 , Article number: 47 ( 2021 ) Cite this article
32k Accesses
17 Citations
15 Altmetric
Metrics details
A Correction to this article was published on 17 June 2021
This article has been updated
Key message
The history of the relationship between plant growth and water consumption is retraced by following the progression of scientific thought through the centuries: from a purely philosophical question, to conceptual and methodological developments, towards a research interest in plant functioning and the interaction with the environment.
The relationship between plant growth and water consumption has for a long time occupied the minds of philosophers and natural scientists. The ratio between biomass accumulation and water consumption is known as water use efficiency and is widely relevant today in fields as diverse as plant improvement, forest ecology and climate change. Defined at scales varying from single leaf physiology to whole plants, it shows how botanical investigations changed through time, generally in tandem with developing disciplines and improving methods. The history started as a purely philosophical question by Greek philosophers of how plants grow, progressed through thought and actual experiments, towards an interest in the functioning of plants and the relationship to the environment.
This article retraces this history by following the progression of scientific questions posed through the centuries, and presents not only the main methodological and conceptual developments on biomass growth and transpiration but also the development of the carbon isotopic method of estimation. The history of research on photosynthesis is only touched briefly, but the development of research on transpiration and stomatal conductance is presented with more detail.
Research on water use efficiency, following a path from the whole plant to leaf-level functioning, was strongly involved in the historical development of the discipline of plant ecophysiology and is still a very active research field across nearly all levels of botanical research.
1 Introduction
The ratio of biomass accumulation per unit water consumption is known today as water use efficiency (WUE) and is widely relevant to agriculture (e.g. Blum 2009 ; Tallec et al. 2013 ; Vadez et al. 2014 ), to forest ecology (e.g. Linares and Camarero 2012 ; Lévesque et al. 2014 ) and in the context of global climate change (Cernusak et al. 2019 ). This ratio can be defined at various levels, from the physiological functioning of a leaf to the whole plant and at the ecosystem level. This historical review starts at the whole plant level, where WUE can be simply measured by quantifying the amount of water given to a plant and the plant’s increase in biomass during the experiment. The ratio of biomass produced divided by the cumulative water lost during growth is termed whole plant transpiration efficiency (TE= biomass produced/water lost). Historically, the ratio has also been calculated in its inverted form (water lost/biomass produced) and various terms have been used to denote these ratios (see Box 1). As knowledge, concepts and technology advanced, it became desirable to measure TE also at the leaf level, where it is defined either as the ratio of net CO 2 assimilation rate to transpiration (or to the stomatal conductance for water vapour). Therefore, some history of the two leaf-level components of WUE is included here. Numerous articles have been published on the history of the development of research on photosynthesis, and other than the reviews cited in this article, the publications by Govindjee are notable, especially Govindjee and Krogmann ( 2004 ), as they include a long list of other writings on the history of photosynthesis. On the other hand, little has been written about the history of research on transpiration and stomatal conductance. Notable is Brown ( 2013 ), who wrote specifically on the cohesion-tension theory of the rise of sap in trees, including many writings from the late nineteenth century. Consequently, here, photosynthesis research is only broached briefly, whereas transpiration research is more detailed.
As the development of the research on WUE spans a very long period, starting with Greek philosophers, publications are in several languages. Classical writings were in Greek or in Latin, and for these translations are available. However, from the mid-seventeenth century onwards, national languages were more and more used, which can be seen in the number of French- and German-language publications. This review is also a tribute to these nowadays less known seventeenth, eighteenth and nineteenth century French and German natural philosophers and their contribution to the development of the science of plant ecophysiology. Also, towards the beginning of the twentieth century, publications became too numerous to allow a comprehensive review; thus, the author focussed on the use of the carbon stable isotopes methodology and on tree ecology.
Box 1 Short history of names for whole plant transpiration efficiency (TE)
2 what is plant matter made of.
Various Greek philosophers were interested in how substances can change from one thing into another. Thales (624–c. 546 BC) thought that all things come from water, whereas Anaximenes argued that “pneuma” (air) should be the basis of all things (Egerton 2001a ). These assertions were the basis of more than 2000 years of philosophical dispute.
In “De Causis Plantarum”, Theophrastos (371–287 BC) assumed that plants draw nutrition, which consisted of varying amounts of the four elementary humours, from the earth through their roots (Morton 1981 ). Some centuries later, in a Christian work translated in 400 AD from Greek into Latin and known as “Pseudo-Clement’s Recognitions”, an apparent thought experiment was described to “prove that nothing is supplied to seeds from the substance of the earth, but that they are entirely derived from the element of water and the spirit (spiritus) that is in it” (Egerton 2004c ). The author of this thought experiment suggested putting earth into big barrels, growing herbaceous plants in it for several years, then harvesting them and weighing them. His hypothesis was that the weight of the earth would not have changed, and the author used this as an argument that the vegetation biomass could have come only from water. This thought experiment revealed a progress in scientific thinking because the question was posed more precisely than before. It stood out at a time when botany mainly consisted of naming plants and “theoretical botany effectually went out of existence” (Morton 1981 ).
It appears that the question of how plant matter is produced was not pursued in Roman or Arabic writings, which were more concerned with agricultural (the former) and medical (the latter) aspects of plant sciences (Egerton 2001b , 2002 ). Not until the High Middle Ages was a renewed interest shown in plant growth. Adelard of Bath, a twelfth century English natural philosopher, devoted the first four chapters of “Questiones Naturales” (c. 1130–1140; Morton 1981 ) to the question of what plant matter is made of. He argued, within the concepts of the four elements theory, “by just as much as water differs from earth, by so much does it afford less nourishment to roots, I mean than earth does”, clearly being in favour of earth as the source for plant nourishment. His arguments were only theoretical and speculative.
A major step occurred in botanical sciences between the fifteenth and sixteenth centuries; scholars began making experiments to test antique and medieval hypotheses against observations in nature (Egerton 2003 ). In the mid-fifteenth century, and probably related to the translation and printing of the botanical books by Theophrastus (Morton 1981 ), the thought experiment from “Recognitions...” was taken up by Nicholas of Cusa in the fourth part of his “Idiota de mente”, “De staticis experiments”. At a time when the naming of plants for pharmacology was the major interest of savants, he proposed experimental investigations. Nicholas of Cusa described the same thought experiment as did Pseudo-Clement’s Recognitions ; he concluded similarly that “the collected herbs have weight mainly from water” (1450; translation into English by Hopkins 1996 ). Cusa additionally suggested that the plants should be burned at the end of the experiment and the ash weight be taken into account. It is not clear whether the thought experiment was ever physically done.
In the sixteenth century, botanical science began to separate from medical sciences, with the establishment of lectureships in universities (e.g. Padua in 1533) and the establishment of botanical gardens (Egerton 2003 ). The bases existed for advancing science in the seventeenth century of Enlightenment. Francis Bacon, an influential philosopher of his time, conducted a series of plant growth experiments which are reported in his “de Augmentis Scientiarum” (1623; Spedding et al. 1900 ). Bacon discovered that some plants sprouted more quickly in water than in soil (Egerton 2004b ). He concluded that “for nourishment the water is almost all in all, and that the earth doth but keep the plant upright, and save it from over-heat and over-cold” (Hershey 2003 ), thus still upholding the theory proposed by Thales and Nicholas of Cusa. In “The History of the Propagation and Improvement of Vegetable”, Robert Sharrock ( 1660 ) reported that some plants both rooted and grew entirely in water. Although he noted different amounts of transpiration over time, he did not discuss this in relation to plant growth.
In 1662, Johannes Baptista van Helmont published his now-famous willow experiments (van Helmont 1662 ). This may be the first report of an experiment that was based on the thought experiment of Nicholas of Cusa (Hershey 2003 ) with the minor differences of beginning with dried soil and not using herbaceous plants, but rather a willow tree. After weighing the soil, he irrigated it with rain water and planted the weighed stem of a willow tree. The experiment ran for 5 years. At the end, the tree was weighed again, as was the dried soil. He found the soil weighed about 2 ounces less than at the beginning of the experiment, whereas 164 pounds of wood, bark and roots was produced. He concluded that the organic matter could only have come out of the water. Helmont was unaware of the existence of carbon dioxide, but he did know of “gas sylvestre”. He also knew that burning oak charcoal would produce nearly the same amount of gas sylvestre and ash. However, he did not connect this information with the plant growth he had observed (Hershey 2003 ). Robert Boyle published similar experiments in “The sceptical Chymist” (Boyle 1661 ). Boyle claimed that he had done his experiments before he knew of Helmont’s (Egerton 2004c ), although he discussed Helmont’s results and arguments in detail in his book. Boyle doubted the direct transformation of water into plant matter. He admitted, however, that it might be possible that other substances contained in the water could generate new matter (Boyle 1661 ). In the 1660’s, Edme Mariotte also criticised van Helmont’s theory that water alone constituted the only element to produce plant matter. He thought similarly to Boyle that elements in the water could contribute to the plant matter. He also showed that nitrogen compounds were important for plant growth (Bugler 1950 ).
John Woodward, in his “Some Thoughts and Experiments Concerning Vegetation” (Woodward 1699 ), took up again the question of what comprised the source of plant growth. Woodward criticised Helmont’s and Boyle’s experiments, mainly on the precision of weighing the dry soil before and after the experiment, but also the contamination of the irrigation water by terrestrial vegetable or mineral matter. Consequently, he developed a series of hydroponics experiments, where by growing plants in sealed vials, in different types of water and weighing them regularly over the same time period, he could calculate how much biomass was gained over a set time period. He was able to draw a series of conclusions from these experiments by calculating the ratio of water lost to plant mass gained in the same period of time, thereby calculating the inverse of transpiration efficiency. This was probably the first time that the inverse of transpiration efficiency was calculated using experimental data. He showed that 50 to 700 times as much water was lost than biomass gained. He also reported that plants grown in water containing more terrestrial matter grew more and with less water consumed. From these observations, he concluded that water serves only as a vehicle for the terrestrial matter that forms vegetables and that vegetable matter is not formed out of water. He is still remembered more for his geological publications (Porter 1979 ) than for his contributions to botany (Stanhill 1986 ).
In his “history of ecology” series, Egerton ( 2004c ) nicely sums this period thusly: “each of these authors (Bacon, Boyle, Helmont, Sharrock) built upon the work of his predecessors and improved somewhat the understanding of plant growth and how to study it. However, they still fell short of a basic understanding of plant growth. Before that could be achieved, chemists would have to identify the gases in the air”. This series of studies shows that from the end of the seventeenth century onwards, experiments replaced speculation (Morton 1981 ), in botany as well as in many other areas of science.
From the end of the seventeenth century, the question of how plants grow was still unresolved, although it was known that nutrients were conducted from the roots in the ascending sap to the leaves. A major improvement in the understanding of how transpiration and its variations work was the discovery of cells by Robert Hooke towards the middle of the seventeenth century (Egerton 2005 ) and subsequently the discovery of stomata on leaf surfaces. One of the first to describe stomata may have been Malpighi in “Anatomy of Plants” (Malpighi 1675 in Möbius 1901 ). Based on Malpighi’s and Grew’s ( 1682 ) studies, John Ray suggested in “Historia Plantarum” (Ray 1686 in Lazenby 1995 ) that the apertures in the leaves, when open, would give off either breath or liquid. Ray may have been the first to have connected stomata with transpiration. He also suggested that the loss of water by evaporation is compensated constantly by water from the stem, and thus transpiration results from a constant water flux. He also observed that sap ascends the stems of trees in sap-bearing vessels which do not contain valves. He did, however, admit that it cannot be capillary forces that make water go up tall trees.
Ideas on photosynthesis developed slowly from the middle of the seventeenth century onwards. Malpighi ( 1675 ) suggested that leaves produce (“concoct and prepare”) the food of plants and from leaves this food passes to all parts of the plant. Similarly, Claude Perrault in “Essais de Physique” (Perrault 1680 ) defended the hypothesis that the root acts as the mouth of the plant and that the leaves serve to prepare the food arriving with the sap from the root so that it can be used in the rest of the plant. John Ray in “History Plantarum” (Ray 1686 in Lazenby 1995 ) concurs with this, however adding in “The wisdom of God” (Ray 1691 in Lazenby 1995 ) that “not only that which ascends from the Root, but that which they take in from without, from the Dew, moist Air, and Rain”. He also thought that light could play a role in this preparation of the plant sap. At this time, most authors (Malpighi, Perrault, Mariotte, Ray) knew about the circulation of sap, up as well as down, and that leaves served somehow to transform the upcoming sap into food for the plant.
In 1770 , Lavoisier published “Sur la nature de l’eau” (“On the nature of water”, translation by the author) and reviewed the literature on the possibility of water changing into earth to nourish plants. Lavoisier cited the Van Helmont experiment and later works which tested Van Helmont’s idea by growing plants in water (e.g. Boyle, however he did not cite Woodward). He was critical of the idea that it could be a transformation of water that would constitute plant material. This was based mainly on experiments by himself and others, showing even distilled water would contain traces of “soil”. However, he also defended the idea, based mainly on Charles Bonnet’s observations, that leaves absorb vapours from the atmosphere that contribute to plant growth.
Helmont had coined the term “gaz” in the mid-seventeenth century and had been able to distinguish different gazes from air (Egerton 2004a ). It was only in the middle of the eighteenth century that gases were studied in the laboratory and several observations by different researchers would finally lead to an understanding of respiration and photosynthesis (Tomic et al. 2005 ; Nickelsen 2007 ). Richard Bradley seems to be one of the first to clearly state (in letters from 1721 to 1724) that plant nourishment can be drawn from the air. Hales ( 1727 ) agreed with this theory, which was not yet widely accepted (Morton 1981 ), and suggested that light might be involved, which helped to pave the way for the discovery of photosynthesis. Black ( 1756 ) was able to identify carbon dioxide (which he called fixed air) using a lime water precipitation test. He demonstrated that this “fixed air” did not support animal life or a candle flame (Egerton 2008 ). Charles Bonnet ( 1754 ) made an important observation, i.e. branches with leaves that were submerged under water would produce air bubbles on their surfaces when sunlight shone on them, but not after sunset. Senebier refined these experiments in 1781 (Morton 1981 ), by showing that the leaves produced no oxygen in the sunlight when the surrounding water was free of carbon dioxide and that the rate of oxygen production was higher with carbon dioxide-saturated water. Tomic et al. ( 2005 ) present nicely the steps leading up to the term photosynthesis. This began with Priestley ( 1775 ) demonstrating that the air given off by animals and by plants was not the same, Ingen-Housz ( 1779 ) observed the important role of light, and the dispute between Senebier and Ingen-Housz from 1783 to 1789 resolved more clearly the functions of carbon dioxide emission (respiration) and absorption (photosynthesis). Based on these results and his own very detailed observations, de Saussure reported in 1804 that the carbon necessary for plant growth is absorbed mainly by green leaves from atmospheric carbon dioxide and he estimated that the largest part of the accumulated dry matter of plants is made of this carbon. Thus, the dispute of what the plant matter is made of that began in antique Greece was resolved at the end of the eighteenth century.
3 How much water do plants need to grow?
The late eighteenth century marked the beginning of applied agricultural science and the rise of plant physiology (Morton 1981 ). Work continued on transpiration and stomata, with a large number of experiments. Burgerstein ( 1887 , 1889 ) managed to assemble 236 publications on transpiration of plants from 1672 to 1886, citing short abstracts of each and comparing them critically. Also, Unger published in 1862 a major review article covering such subjects as the relationship of transpiration to temperature and humidity; daily cycles, including night; differences in adaxial and abaxial leaf surfaces; the impact on transpiration of type, number, size and distribution of stomata; the structure of the epidermis (cell layers, cuticle, hairs and wax); development of the mesophyll; size of intercellular spaces and cell turgor; and the impact of plant transpiration on the atmosphere (Unger 1862 in Burgerstein 1887 ). Scientists started to reflect on the interaction of plants, or more specifically their leaves, with their environment, and experimentation included the responses of stomata to light quantity (Möldenhawer 1812 ) and quality (Daubeny 1836 in Burgerstein 1887 ). Based on inconsistent observations by e.g. Banks, Möldenhawer and Amici, advances were also made on the functioning of stomata (Mohl 1856 ). However, progress was mainly based on a comment in von Schleiden ( 1849 ) that the state of the stomata would be the result of the water in- or outflow of the pore cells (called “Schliesszellen”) and he showed experimentally that stomata close when the pore cells lose water. As knowledge of transpiration, stomatal opening and their dependence on environmental variables increased, new questions arose about the water consumption of plants.
Another milestone along the way to understanding the transpiration of plants in the nineteenth century was the publication by Sir John Bennet Lawes ( 1850 ), “Experimental investigation into the amount of water given off by plants during their growth; especially in relation to the fixation and source of their various constituents”. He described experiments on wheat, barley, beans, peas and clover using differently fertilised soils. He was using plants in closed containers and an especially designed balance to “estimate the amounts of water given off” (Fig. 1 ). He observed increased evapotranspiration with higher temperatures during the growing season, and asked whether “this increased passage of water through the plants, carrying with it in its course many important materials of growth from the soil, and probably also influencing the changes in the leaves of these, as well as of those derived from the atmosphere, will not be accompanied with an equivalently increased growth and development of the substance of the plant”. This was followed by an important discussion of the influence of temperature on evaporation and growth as well as the resultant ratio. He discussed in the introduction “the relationship of the water given off to the matter fixed in the plants”; he gave his results in this ratio and in the inverse ratio, and applied these ratios to different scientific questions. The first ratio (transpired water divided by plant matter, the inverse of today’s TE) was used to interpret his results in terms of water use compared to field available water, and the latter’s ratio (plant matter divided by transpired water, equivalent to today’s TE) was used to discuss his results in terms of functional differences among species. From the observed functional differences, he concluded that there was “some definite relationship between the passage of water through the plants and the fixation in it of some of its constituents”. He was, thereby, introducing a new question about the link between dry matter accumulation and transpiration, which will be treated in the next chapter.
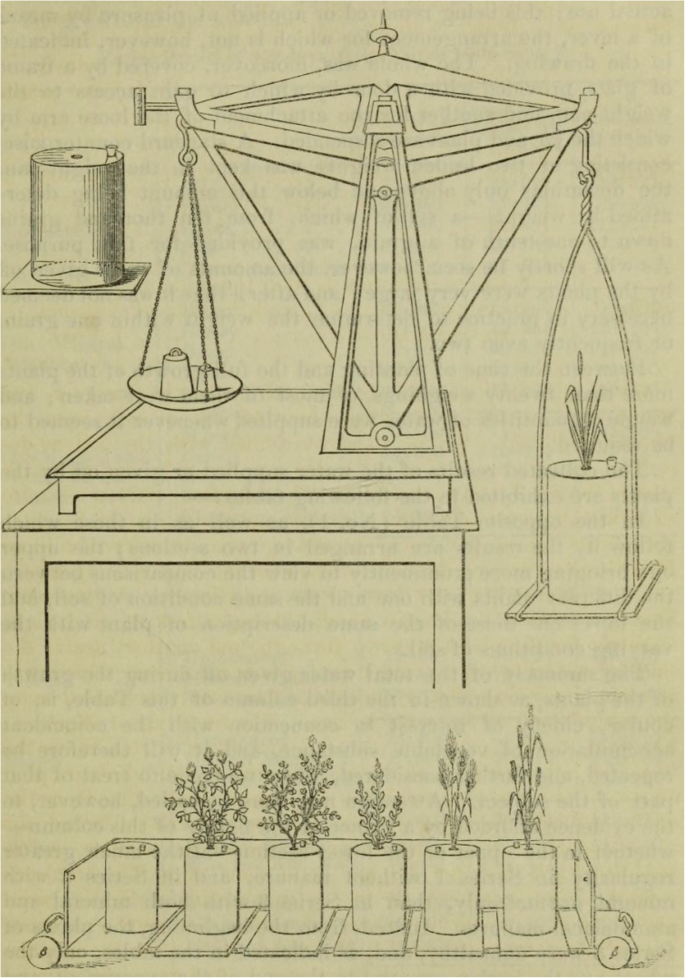
Illustration from Lawes ( 1850 , p. 43) of the special balance constructed for weighing plants in their “jars” to estimate the amounts of water given off and also the “truck” on which a series of jars was moved to the balance
Towards the end of the nineteenth century, research interest started to include agricultural questions of water use. Marié-Davy ( 1869 ) measured transpiration (standardised by leaf surface) of over 30 plant species, including eight tree or shrub species as well as herbaceous and agricultural plants. He estimated transpiration per soil area, thereby establishing that a prairie would transpire more than trees. von Höhnel ( 1879 ) estimated long-term transpiration of branches of 15 tree species (standardised on leaf surface or leaf dry weight). He used these data of branch transpiration to upscale to whole trees and concluded that compared to agricultural plants, the amount of rain seemed sufficient for tree growth. Hellriegel ( 1871 ) had already similarly concluded for cereals in the Mark Brandenburg (Germany) region that rainfall would not be sufficient, as had Marié-Davy ( 1874 ) for wheat in the Paris (France) region. In parallel with these more quantitative interrogations about water use, from the mid-nineteenth century, scientists started to ask more functional questions about the relationship between transpiration and dry matter accumulation, in a context of vigorous growth of botanical sciences and the complex relation between organisms and their environment (Morton 1981 ).
4 Are transpiration and dry matter accumulation linked?
Lawes ( 1850 ) had already reflected on a functional relationship between water flux and plant matter accumulation. In the following years, there were several publications on the transpiration of trees, and although no transpiration efficiency was estimated, the understanding of tree transpiration advanced. Many comparative studies were published. Lawes ( 1851 ) on “Comparative evaporating properties of evergreen and deciduous trees” considered twelve different tree species. He provided measurements of the variation in transpiration with temperature and hygrometry data. With these, he concluded that “evaporation is not a mere index of temperature but that it depends on vitality influenced by heat, light and other causes”. In the late nineteenth century, several researchers estimated and compared values of the ratio of transpiration and dry matter accumulation for different plants (Burgerstein 1887 ). With the growing evidence of variation in this ratio, scientists started to reflect on the relationship between transpiration and dry matter accumulation, aided by the development of new measurement techniques. A major question was if there would be a tight coupling between transpiration and dry matter accumulation, resulting in a constant transpiration efficiency, or if variation could be observed.
Dehérain ( 1869 ) studied evaporation and the decomposition of carbonic acid in leaves of wheat and barley. Using an ingenious apparatus, he was probably the first to directly measure evaporation of water in parallel with carbonic acid decomposition. He studied the effect of variously coloured light, and although he did not calculate the ratio between evaporation and carbonic acid decomposition, he did conclude that light of different colours had a similar effect on carbonic acid decomposition and on water evaporation from the leaves. His final conclusion was that “it is likely that there is existing between the two main functions of plants, evaporation and carbonic acid decomposition, a link, of which we need to determine its nature” (translation from the original French by the author). Several other scientists also commented on the relationship between transpiration and dry matter production. Fittbogen ( 1871 ) supposed, similarly to Lawes ( 1850 ) before him, but with more experimental evidence, that there should be a positive relationship between transpiration and production of dry matter. Dietrich ( 1872 in Burgerstein 1887 ) supposed that this relationship would be linear, whereas Tschaplowitz ( 1878 in Burgerstein 1887 ) introduced the idea that there should be an optimum transpiration at the maximum production of matter. Therefore, when the transpiration would increase over this optimum, this would lead to a decrease in assimilation rate. He was one of the first to suggest a non-linear relationship between transpiration and assimilation. Sorauer in “Studies on evaporation” ( 1880 ) defended the hypothesis that transpiration was not only a physical phenomenon but was also physiological. He stated that “It is not possible as yet to study the plant internal processes which regulate the transpiration, however it is possible to quantify the relationship between dry-matter and transpiration” (translation from German by the author), suggesting thereby TE as a means to advance the understanding of plant internal processes. Sorauer was probably at the cutting edge of science of his time. He pointed out specifically that variability among plants of one species was due to genetics (German, “erbliche Anlagen”), a startling and even daring assertion for his time. He asserted that for comparative studies, genetic variability needed to be minimised. To achieve this, he used, when possible, seeds from the same mother plant, grown in the same environmental conditions and a large number of repetitions. Using these protocols, he was probably one of the first to estimate TE on tree seedlings, showing that there was within species diversity in transpiration and growth, but that their ratio was more constant. He concluded from experiments on pear and apple trees that the pear trees used less water for the same biomass growth. He was able to go one step further and demonstrate that this difference was due to less transpiration per leaf area. By comparing different woody and herbaceous plants with different growth types, he postulated that when plants had a small leaf area combined with high transpiration, they had either a very strong growth increment, a high dry matter percentage, or a large root system. Overall, he observed relationships between dry matter production and transpiration; he concluded that there must be some regulation of the transpiration per unit leaf area by the co-occurring dry matter production.
Hellriegel ( 1883 ) argued that one cannot estimate a constant ratio between transpiration and production as there were factors which influence each independently. He also commented that it might make sense to estimate mean values of transpiration for various agricultural plants, as this would be for practical and scientific value. He thought that the most logical standardisation would be by the mass of the dry matter produced during the same time period. He called this “relative Verdunstungsgrösse” which can be translated into English as “relative transpiration”. He was probably one of the first to give a name to the ratio between whole plant transpiration and dry matter production. He proposed a theory that for a long-term drought, plants would acclimate their morphology to decrease their “relative transpiration”. He provided additional experimental evidence that barley had decreased in relative transpiration over as many as seven levels of soil water deficit, relative to field capacity. Using his own observations, he proposed that when calculating a mean “relative transpiration” for a single species, variation of transpiration should be minimised and that plants should be tested together only under optimal conditions. Given the relatively small differences in relative transpiration that he observed among different crops, Hellriegel suggested that these differences would not explain why some crops grow better in wet locations and others on dry locations. Hellriegel was thus probably one of the first scientists to point out that the relationship between drought adaptation and “relative transpiration” might not be straightforward.
Understanding how biomass and water loss were connected was studied by Iljin ( 1916 ) on a newly detailed level. He measured simultaneously water loss and carbon dioxide decomposition and reported his data as grammes of water lost per cubic centimetre of carbon dioxide decomposed. He concluded from studying more than 20 plant species that “...it is generally agreed that the rates of water loss and of CO 2 assimilation are directly proportionate to stomatal aperture, and that consequently there exists a close connection between these two processes”.
At the end of the nineteenth century, the ratio of transpiration versus dry matter accumulation was recognised as an important plant trait, which varies among and within species in a complex interaction of each component with the other and with environmental factors.
5 How do plants differ in water requirement and how do they respond to variations in environmental factors?
In the late nineteenth century, several researchers estimated and compared values of the ratio of transpiration and dry matter accumulation for a range of cultivated plants (Fittbogen 1871 ; Dietrich 1872 ; Farsky 1877 , cited in Burgerstein 1887 ), giving evidence of the growing interest of agricultural scientists. The number of studies of transpiration efficiency greatly increased, thereby driving a new standardisation in terminology. King ( 1889 ) studied the inverse of transpiration efficiency and described it as “the amount of water required for a ton of dry matter”, and promulgated this terminology by using it in the titles of his publications between 1892 and 1895. Similarly, Leather ( 1910 ) published “Water requirements of the crops of India”, in which he defined the “transpiration ratio” as “the water transpired to the weight of dry plant produced”. The shift from a purely descriptive use of “water requirement” to a clearly defined one was provided by Kearney and Shantz ( 1911 ) as “… the degree to which a plant is economical in its use of water is expressed in its water requirement, or the total quantity of water which it expends in producing a pound of dry matter”. The term “water requirement” is the inverse of the modern transpiration efficiency, and was used by a rapidly increasing number of publications which were published on the water use of crops in the early twentieth century. Montgomery ( 1911 ) may have been the first to use the term for a plant trait in “Methods of determining the water requirements of crops”.
At the beginning of the twentieth century, the importance of gaining knowledge on the water requirements of plants can be seen in the technical effort that went into the measuring equipment. von Seelhorst ( 1902 ) presented a system of growing boxes on rails, placed belowground, including the balance, so that the top of the growing boxes was at the same level as the surrounding soil (Fig. 2 ). In the now well-known studies on “The water requirement of plants. I. Investigations in the Great Plains in 1910 and 1911”, Briggs and Shantz ( 1913a ) measured the water requirement for 21 crop and weed species, sometimes for different varieties of the same crop and under controlled and field conditions. In the same year, they reviewed the available literature on water requirement (Briggs and Shantz 1913b ), increasing their dataset to 31 different crop species. They discussed in detail studies from 29 different authors, many of which had only published once or twice on this subject. A few researchers were notable for their number of publications on the water requirement of crop plants: King with 6 publications between 1889 and 1905, and von Seelhorst with 9 publications between 1899 and 1907. The largest contributions came from Hellriegel ( 1883 ; 10 species) and Leather ( 1911 ; 15 species). Kiesselbach ( 1916 ) also reviewed 59 publications from 1850 to 1915 “which had studied transpiration in relation to crop yield, based upon plants grown beyond the seedling stage”. There were regular publications of original work from 1870s onwards, with more than one publication per year from 1890 onwards. The difference among species and the impact of environmental factors on water requirement was one of the main questions raised. These reviews and the increasing amount of newly published work per year are evidence of the growing interest in the “water requirement” of plants as a trait of increasing importance in agricultural sciences.
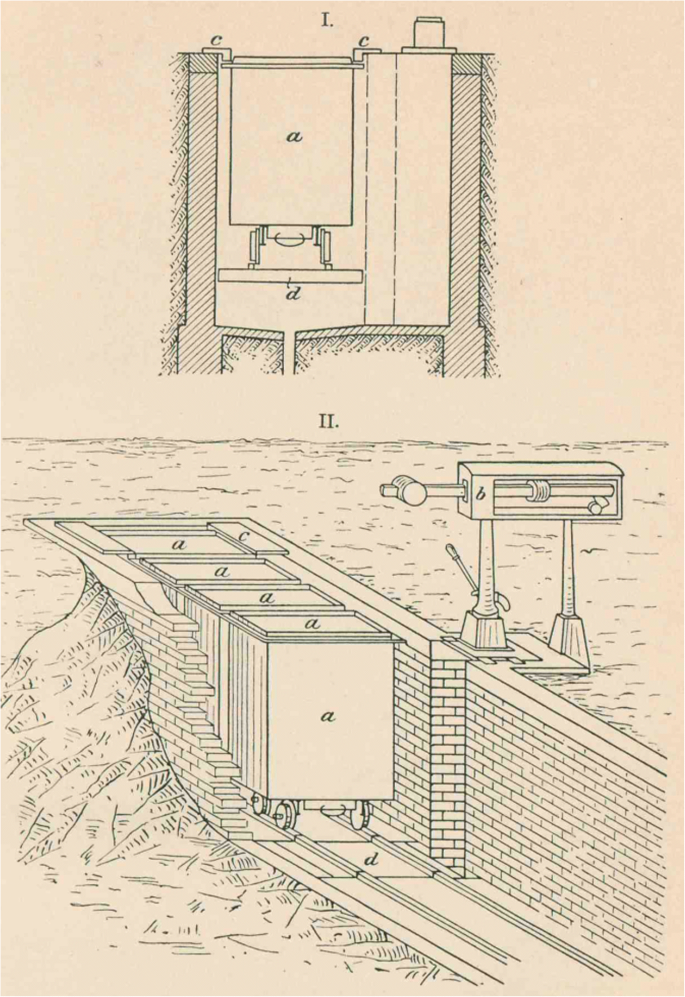
Illustration from von Seelhorst ( 1902 ), showing the quite sophisticated outdoor installation “Vegetationskasten” (growing boxes, translations by the author) to weigh plants in small waggons, with a “Kastenwagen” (boxwaggon), b “Waagebalken” (scale beam), c “Deckbretter” (cover board) and d “Waagentisch” (weighing table)
With regard to species differences in water requirement among crops, Schröder ( 1895 , cited in Maximov 1929 ) found two groups, among seven cereals, which differed in water requirement by a factor of 2. Millet, sorghum and maize were known to be drought resistant, and showed a lower water requirement than the remaining plants. These differences were confirmed by Kolkunov ( 1905 , cited in Maximov 1929 ), Briggs and Shantz ( 1914 ), Briggs and Shantz ( 1917 ) and Shantz ( 1927 ). Millet, sorghum and maize are now known to use the C4 carbon pathway of photosynthesis.
With regard to external environmental influences on plants, Briggs and Shantz ( 1913b ) distinguished between soil, atmosphere and plant factors. Soil factors which were investigated were soil moisture content, soil type, cultivation, soil volume, soil temperature, effect of fertilisers in soil or water cultures and effect of previous crops. Weather factors considered were air temperature and humidity, shade and carbon dioxide content. Other factors studied in direct relationship to the plants were parasite attacks, relative leaf area, cutting frequency, defoliation, planting density and the age of plants.
A critique of the term “water requirement” was not long in coming. Dachnowski ( 1914 ) wrote, “It is assumed by many writers that a definite and quantitative relation exists between transpiration and growth, and that hence the ratio of the weight of water absorbed and transpired by a plant during its growth to the green or dry substance produced is an adequate and simple measure of growth.”, followed by an argument why this was not the case.
6 Why do plants differ in transpiration efficiency?
The adaptations of plants to dry environments were an important ecological topic at the beginning of the twentieth century, as the discipline of “physiological ecology” (Iljin 1916 ; Moore 1924 ) began to develop. Iljin ( 1916 ) studied more than 20 different plant species in situ from different ecological locations, e.g. wet bottom soils and variously facing slopes of ravines with different aspects. Iljin proposed that “the water requirements of the different species should be very different, and consequently the amounts of water available should differently affect their processes of life”. Using his observations, he was able to show that “… in no case was the water loss per unit of decomposed CO 2 found to be equal to or more in xerophytes than in mesophytes”, thus suggesting a higher transpiration efficiency. He argued that mesophytes would have to close stomata “… in dry places in order to reduce evaporation, thus diminishing the rate of assimilation as well, whereas in the case of xerophytes, which are adapted to extreme conditions of existence, assimilation in similar circumstances proceeds actively”. He then tried to confirm his hypothesis by transplanting mesophytes from wetter sites to the drier environment of xerophytes. Iljin showed experimentally that in all cases, a higher water requirement was measured for mesophytes transferred to a drier site compared to their original site and compared to xerophytes at the dry site. He interpreted his observations as “plants growing in dry places are adapted to a more economical consumption of water”. He held this to be true for among- and within-species variation.
A milestone in forest “physiological ecology” was Bates’ ( 1923 ) study of the physiological requirements of Rocky Mountain trees. Bates wrote that for foresters, knowledge of demands of tree seedlings for moisture, light, heat and soil fertility was important for planning reforestation. He started a large investigation of six forest tree species, combining field studies to describe ecosystems, with experiments in controlled environments in order to determine species differences in relative transpiration and other water flow-related traits. Bates concluded from the comparison among species that trees of low water requirement would be trees that have a superior control over their water supply. He was however critical of a direct relationship between water requirement and drought resistance in trees. Moore ( 1924 ) commented that in correlating physiological measurements with the habitat characterisation of the species, Bates “... has opened new fields to forest investigations”. He also stressed that the results were counterintuitive in that the most xerophytic species had the highest water requirement, whereas the most mesophytic species had the lowest water requirement.
A similar discrepancy was observed by Maximov ( 1929 ) in the chapter “Efficiency of transpiration” in his book The Plant in relation to water , which was translated from Russian into English rapidly after its publication. Maximov preferred “efficiency of transpiration” to “water requirement”, arguing that the former would be more logically correct, because the determining process (transpiration) should be in the denominator, which also would have the effect that “… an increase in the figure denoting the value of the ratio actually corresponds to an increase of the efficiency per unit of water used”.
In his book, Maximov ( 1929 ) described experiments done at Tiflis Botanic garden (today in Georgia) by Maximov and Alexandrov ( 1917 ), where they studied local xerophytes for 3 years. They found xerophytes with a high efficiency of transpiration, particularly drought-resistant annuals. They also found that plants with a low efficiency of transpiration appeared to be the most typical semi-arid xerophytes. The mesophytes all displayed a medium efficiency. Maximov noted from other observations on the same plants that the “… majority of xerophytes with a low efficiency of water expenditure possess very extensive root systems, far exceeding in length the sub-aerial portions of the plant”. He also observed that these plants showed a strong transpiration and that this transpiration might constitute the “pump” which could draw water through such an extensive root system. He also observed that “members of the group of annual xerophytes with a high efficiency of transpiration are characterised by a relatively large leaf surface, which develops very rapidly”. He argued that this would confer a high intensity of assimilation. From these observations, he concluded a “lack of direct proportionality between efficiency of transpiration and the degree of drought resistance”, but also that “the magnitude of the efficiency of transpiration affords one of the most satisfactory tests of the ecological status of a plant”. Maximov applied the ecological classification developed by Kearney and Shantz ( 1911 ), which they had based on plants of the arid and semi-arid regions of North America: (1) drought-escaping with an annual growth cycle restricted to favourable conditions; (2) drought-evading, delay by various means the exhaustion of soil moisture; (3) drought-enduring, can wilt or dry but remains alive; and (4) drought-resisting, can store a water supply. It should be noted that the ecological definitions behind these concepts have changed with time and are used slightly differently today. Shantz ( 1927 ) argued that many of the drought-evading plants had a low water requirement and Maximov noted that this group included the highly efficient xerophytes with a large leaf area. Maximov also observed that xerophytes from the third group (drought-enduring) could show a very low efficiency of transpiration and belonged to the group of xerophytes with large root systems. Without concluding directly, he suggested a relationship between the transpiration efficiency of a xerophyte and its ecological strategy when facing limited soil water content. These studies by Maximov are among the most complete concerning the relationship between a plants’ resistance to drought and their transpiration efficiency, reflecting the interest of scientists in ecological questions of plant functioning, especially in relation to drought.
Although work on crop plants advanced greatly in the early twentieth century, results were scarcer for tree species. Raber ( 1937 ) concluded his book on “Water utilization by trees, with special reference to the economic forest species of the north temperate zone” with detailed discussions of available data for forest trees. He commented that “much more work on the water requirements of trees of all ages and under varying site conditions is needed”. And he continued that “In view of the importance of planting drought-resistant species in regions where the water supply is below the optimum for most tree species, it is extremely urgent to know more about what qualities make for drought resistance and what species possess these qualities to the greater degree.” These conclusions by Raber show that from the beginning of the twentieth century, the estimation of transpiration efficiency had taken an important place in ecological studies on forest tree species, however not without some critical thoughts on the subject.
7 What is the functional importance of transpiration?
Already in the 1870s and 1880s, the role of stomata in the diffusion of carbon dioxide into the leaf (during the day) and out of the leaf (during the night) was discussed in the scientific literature, as shown by the extensive literature review by Blackman ( 1895 ) (see also section 4 above). Especially the functional importance of transpiration was an open question. There were two opposing lines of thought. As summarised by Iljin ( 1916 ), one defended the line of inquiry that transpiration was important only in the process of transporting mineral salts from roots to leaves; the other held that the opening of stomata was necessary for absorbing the carbonic acid from the atmosphere, which leads to a loss of water and is described as an “inevitable evil”. Iljin ( 1916 ) preferred the second line of investigation and attributed a major role to the stomatal aperture, which controlled both the absorption of carbonic acid from the atmosphere and the loss of water. He concluded that in “physiologico-ecological” investigations, assimilation should be studied together with transpiration. Maskell published a series of papers in 1928, where especially “XVIII.—The relation between stomatal opening and assimilation.” (Maskell and Blackman 1928 ) used an apparatus to estimate apparent CO 2 assimilation and transpiration rate in parallel (Fig. 3 ), and was therefore able to study in detail their interdependence, developing the first mathematical descriptions, based on the development of the theories about the diffusion of gases (Brown and Escombe 1900 ). Methodological advances intensified research on the leaf-level relationship between assimilation and transpiration and allowed the study of plant functioning in more detail. The major step forward was the construction of an infrared gas analyser (URAS: in German “Ultrarotabsorptionsschreiber”, IRGA, infrared gas analyser) by Lehrer and Luft in 1938 (Luft 1943 ) at a laboratory of BASF, IG Farbenindustrie. Normally used in industry and mining, Egle and Ernst ( 1949 ) may have been the first to describe the use of the URAS for plant physiological measurements. By 1959, the URAS was routinely used for measuring stomatal resistance or transpiration in parallel and simultaneously with CO 2 assimilation, on the same leaf (Rüsch 1959 ). This was a great improvement on previous methods and led rapidly to a set of equations for calculating assimilation and stomatal conductance (Gaastra 1959 ).
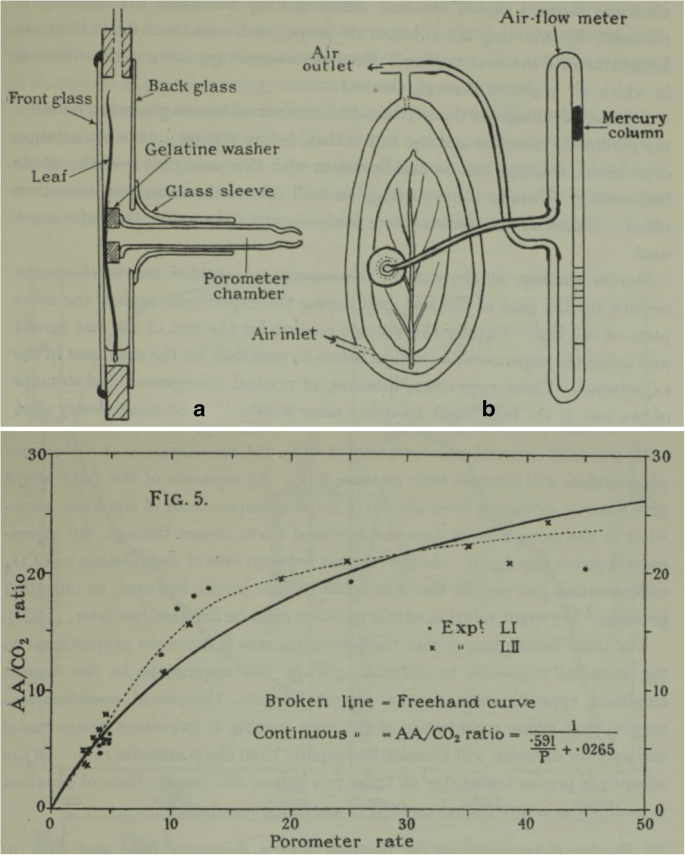
Two figures taken from Maskell and Blackman ( 1928 ): on the top, Figure 1 (p. 489) showing a “Combined assimilation chamber and porometer for simultaneous investigation of apparent assimilation and stomatal behaviour. A. Section of leaf chamber passing through porometer chamber. B. Back view of leaf chamber showing also air-flow meter attached by pressure tubing to porometer and to leaf chamber”. On the bottom, Figure 5 (p. 497) “Relation between porometer rate and apparent assimilation at ‘high’ light, December 1920.” Exp t LI and LII correspond to 2 days of continuous measurements to what Maskell called “diurnal march”
Scarth ( 1927 ) argued that there would be little advantage for a plant to have a high rate of transpiration, but stressed the “... advantage of maintaining the fullest diffusive capacity of the stomata and the highest possible pressure of CO 2 in the intercellular spaces”. He concluded that the principal function of stomata “... is to regulate that very factor which is presumed to regulate them, viz. the concentration of CO 2 in the leaf or, respectively, in the guard cells”. Maskell and Blackman ( 1928 ) tested this hypothesis experimentally and concluded that the rate of uptake of carbon dioxide was determined by variations in stomatal resistance and by resistances within the leaf, thereby introducing the importance of the CO 2 concentrations in the chloroplasts. The suggestion of a strong link between the leaf internal carbon dioxide concentration and leaf-level WUE represented a large advance in the theoretical understanding of WUE.
Penman and Schofield ( 1951 ) proposed, perhaps, the first theoretical link between the leaf-level transpiration ratio (leaf transpiration divided by assimilation) and the ratio of the coefficients of diffusion of water vapour and carbon dioxide in air, and the water vapour and carbon dioxide air-to-leaf pressure gradients. Gaastra ( 1959 ) suggested that the leaf internal conductance to carbon dioxide is a pivotal point of the ratio of assimilation to transpiration and of the water economy of crop plants. Bierhuizen and Slatyer ( 1965 ) showed that the transpiration ratio could be predicted from water vapour and carbon dioxide gradients over a range of light intensities, temperatures and relative humidities. These studies were the first to suggest that whole plant transpiration efficiency might be regulated directly by leaf functioning and would be therefore a trait in its own right and not only the ratio of two plant traits.
8 How can the transpiration ratio be improved?
Because water is increasingly scarce in a warming world, Rüsch ( 1959 ) queried whether the luxury of highly transpiring tree species could be justified. He argued for selective breeding of tree species varieties with low transpiration-to-assimilation ratio T/A by means of minimising transpiration while maximising assimilation. Also Polster et al. ( 1960 ) assessed the potential suitability of tree species to sites by their dry matter production and transpiration ratio. Troughton ( 1969 ) and Cowan and Troughton ( 1971 ) suggested that genetic selection of plant varieties could be used to improve the transpiration ratio by decreasing leaf internal resistance to carbon dioxide diffusion. Cowan and Farquhar ( 1977 ) built on this theme by proposing that stomata might optimise carbon gain to water lost by varying the conductances to diffusion and thereby maximising the ratio of the mean assimilation rate to mean rate of evaporation in a fluctuating environment. Approaches which target photosynthesis, stomatal opening, leaf internal resistance to carbon dioxide diffusion or stomatal optimisation in order to improve plants performance have since been followed in plant breeding and have largely been reviewed elsewhere (e.g. Condon et al. 2004 ; Cregg 2004 ; Vadez et al. 2014 ).
9 Intrinsic water use efficiency and carbon stable isotopes
Another milestone towards contemporary research on water use efficiency was the use of stomatal conductance to water vapour rather than transpiration by Farquhar and Rashke ( 1978 ) and to calculate water use efficiency as assimilation divided by stomatal conductance. This definition allowed an estimation of water use efficiency resulting only from plant functioning, without a direct impact from leaf-to-air vapour pressure difference and was named by Meinzer et al. ( 1991 ) “intrinsic water use efficiency” (W i ). Knowledge of W i facilitated the search for a genetic basis of within species variation, e.g. Brendel et al. ( 2002 ), Condon et al. ( 2002 ) and Chen et al. ( 2011 ).
Development of the stable carbon isotope method for estimating W i resulted in a widely applicable screening method, and a large increase of publications around plant water use efficiency. Based on the two-step fractionation model (atmospheric CO 2 – leaf internal CO 2 – plant carbon) proposed by Park and Epstein ( 1960 ), various models explaining the difference in carbon isotope composition between atmospheric CO 2 and plant carbon were developed in the late 1970s and early 1980s, e.g. Grinsted ( 1977 ), Schmidt and Winkler ( 1979 ) and Vogel ( 1980 ). Vogel’s model contained many theoretical aspects which, however, lacked experimental understanding. In parallel, Farquhar ( 1980 ) developed a similar model, but which resulted in a simple, elegant mathematical equation relating plant natural abundance carbon isotope discrimination, relative to atmosphere, to the ratio of leaf internal to atmospheric CO 2 concentration. This was, in turn, related to W i . Experimental evidence showed that carbon isotope measurements, in wheat, reflected long-term water use efficiency (Farquhar et al. 1982 ) as well as whole plant transpiration efficiency (Farquhar and Richards 1984 ). They concluded that carbon isotope discrimination may provide an effective means to assess and improve WUE of water-limited crops. Strong correlations between whole plant TE and stable carbon isotope measurements of plant organic material were shown in a host of papers to be. Some of these papers were (1) for crops and other annuals (Hubick et al. 1986 ; Ehleringer et al. 1990 ; Virgona et al. 1990 ) and (2) for trees (Zhang and Marshall 1994 ; Picon et al. 1996 ; Roupsard et al. 1998 ). The isotopic method has spread rapidly as a general estimator of WUE and continues to be used widely in screening programmes for plant improvement as well as in ecological research, e.g. Rundel et al. ( 1989 ) and notably used in tree rings (McCarroll and Loader 2004 ).
10 Closing remarks
Water use efficiency is probably one of the oldest of plant traits to stimulate across the centuries the interest of philosophers, theologians, Middle Age savants, natural philosophers and modern plant scientists across different disciplines (plant physiology, ecophysiology, ecology, genetics, agronomy). The interest began as a purely philosophical one, progressed to thought experiments, towards an interest in plant functioning and its relationship to the environment.
Already in the early Renaissance (mid-fifteenth century), an experimentation was proposed, in a time when botany consisted mainly of naming plants (Morton 1981 ). It is then also an early example of an actually performed experimentation, the famous willow experiment by Van Helmont ( 1662 ) as well as of early “in laboratory” experimentation on plants (hydroponics experiments by Woodward 1699 ). The question of what makes plants grow, between water and soil, kept natural philosophers busy up to the end of the eighteenth century, when the assimilation of CO 2 was discovered and the question finally solved.
Early in the nineteenth century, the interest and experimentation turned to the amount of water that plants would need to grow, in the context of a developing research on agricultural practices (Morton 1981 ). Biomass was used to standardise the water losses which allowed comparisons among species (crops as well as trees) and a beginning study of the impact of different environmental variables.
At the end of the nineteenth century, knowledge on the physiological aspects of CO 2 assimilation and the control of transpiration by stomata had sufficiently advanced, so that scientists started to reflect on their inter-dependency. Was transpiration only a physical process or was there a physiological control? Was transpiration regulated by the dry matter production? Or does the stomatal opening determine the rate of CO 2 assimilation?
At the turn of the twentieth century, the study of species differences led to questioning why these differences did exist. As the discipline of “physiological ecology” developed, “water requirement” was inverted into an “efficiency”, reflecting an evolution from standardising transpiration to a trait in its own right. This introduced ecological questions about the adaptation of plants to dry environments and the relation to transpiration efficiency. Counterintuitive results stimulated the discussion and linked differences in WUE to different ecological strategies.
Methodological and theoretical advances in the description of leaf gas exchange in the mid-twentieth century showed the central role of stomata in the control of transpiration and CO 2 assimilation, leading to the idea that stomata might optimise water losses versus carbon gain. The development of carbon stable isotopes as an estimator of leaf-level WUE was an important step not only to further develop these theoretical considerations, but also towards large-scale studies. In parallel, modelling approaches were developed to scale from leaf-level WUE to whole plant TE, e.g. Cernusak et al. ( 2007 ), and to the field or canopy, e.g. Tanner and Sinclair ( 1983 ).
At least from the beginning of the twentieth century onwards, also critical views on the relationship between water requirement and its relation to growth mostly in terms of yield were published (Dachnowski 1914 ). Viets ( 1962 ) asked “Is maximum water use efficiency desirable?”, especially in terms of crop production. Sinclair et al. ( 1984 ) considered different options for improving water use efficiency, however concluding that most of these have important limitations or drawbacks. This discussion is ongoing, as can be seen by the article published by Blum ( 2009 ): “Effective use of water (EUW) and not water-use efficiency (WUE) is the target of crop yield improvement under drought stress”.
Exploration and application of transpiration efficiency at the whole plant level, and its derivatives at other levels, are still a very active research field across nearly all levels of forest science: concerning very rapid processes at the leaf level (Vialet-Chabrand et al. 2016 ), up-to-date genetic and genomic approaches for breeding (Plomion et al. 2016 ; De La Torre et al. 2019 ; Vivas et al. 2019 ), studying local adaptation of trees to their environment in a population genetic context (Eckert et al. 2015 ) or an ecological context (Pellizzari et al. 2016 ), water use efficiency from the plant to the ecosystem (Medlyn et al. 2017 ), estimated at the population level (Rötzer et al. 2013 ; Dekker et al. 2016 ) or modelling up to the global earth level (Cernusak et al. 2019 ), just to name a few. Thus, the first curiosity of Greek philosophers has motivated scientists through history, with many exciting discoveries still to come.
Change history
17 june 2021.
A Correction to this paper has been published: https://doi.org/10.1007/s13595-021-01073-0
Bates CG (1923) Physiological requirements of Rocky Mountain trees. J Agric Res 24:97–164
Google Scholar
Bierhuizen J, Slatyer R (1965) Effect of atmospheric concentration of water vapour and CO 2 in determining transpiration-photosynthesis relationships of cotton leaves. Agric Meteorol 2:259–270
Article Google Scholar
Black J (1756) Experiments upon magnesia alba, quicklime, and some other alkaline substances. Essays Obs Phys Lit 2:157–225
Blackman F (1895) XI. Experimental researches on vegetable assimilation and respiration.—No. II. On the paths of gaseous exchange between aerial leaves and the atmosphere. Philos Trans R Soc B 186:503–562
Blum A (2009) Effective use of water (EUW) and not water-use efficiency (WUE) is the target of crop yield improvement under drought stress. F Crop Res 112:119–123
Bonnet C (1754) Recherches sur l’Usage des feuilles dans les plantes. Elie Luzac, Fils, Göttingen
Boyle R (1661) The sceptical chymist. J. Cadweill for J. Crooke
Brendel O, Pot D, Plomion C, Rozenberg P, Guehl JM (2002) Genetic parameters and QTL analysis of ẟ 13 C and ring width in maritime pine. Plant Cell Environ 25:945–953
Article CAS Google Scholar
Briggs LJ, Shantz HL (1913a) The water requirement of plants. I. Investigations in the Great Plains in 1910 and 1911. US Dep Agric Bur Plant Ind Bull 284:1–48
Briggs LJ, Shantz HL (1913b) The water requirement of plants. II. A review of the literature. US Dep Agric Bur Plant Ind Bull 285:1–96
Briggs LJ, Shantz HL (1914) Relative Water Requirement of Plants. J Agric Res 3:1–64
CAS Google Scholar
Briggs LJ, Shantz HL (1917) The water requirement of plants as influenced by environment. In: Proceedings of the Second Pan American Scientific Congress. Pp 95–107
Brown HR (2013) The theory of the rise of sap in trees: some historical and conceptual remarks. Phys Perspect 15:320–358
Brown H, Escombe F (1900) VIII. Static Diffusion of gases and liquids in relation to the assimilation of carbon and translocation in plants. Philos Trans R Soc B 193:223–291
Brown P, Shrader W (1959) Grain yields, evapotranspiration, and water use efficiency of grain sorghum under different cultural practices. Agron J 51:339–343
Bugler G (1950) Un précurseur de la biologie expérimentale: Edme Mariotte. Rev Hist Sci (Paris) 3:242–250
Burgerstein A (1887) Materialien zu einer Monographie betreffend die Erscheinungen der Transpiration der Pflanzen. Verhandlungen der Zool Gesellschaft Wien 37:691–782
Burgerstein A (1889) Materialien zu einer Monographie, betreffend die Erscheinungen der Transpiration der Pflanzen. II. Theil. Verhandlungen der Zool Gesellschaft Wien 39:399–464
Cernusak LA, Aranda J, Marshall JD, Winter K (2007) Large variation in whole-plant water-use efficiency among tropical tree species. New Phytol 173:294–305
Article PubMed Google Scholar
Cernusak LA, Haverd V, Brendel O et al (2019) Robust response of terrestrial plants to rising CO 2 . Trends Plant Sci 24(7):578–586 1–9
Article CAS PubMed Google Scholar
Chen J, Chang SX, Anyia AO (2011) Gene discovery in cereals through quantitative trait loci and expression analysis in water-use efficiency measured by carbon isotope discrimination. Plant Cell Environ 34:2009–2023
Condon AG, Richards RA, Rebetzke GJ, Farquhar GD (2002) Improving intrinsic water-use efficiency and crop yield. Crop Sci 42:122–131
PubMed Google Scholar
Condon AG, Richards RA, Rebetzke GJ, Farquhar GD (2004) Breeding for high water-use efficiency. J Exp Bot 55:2447–2460
Cowan IR, Farquhar GD (1977) Stomatal function in relation to leaf metabolism and environment. In: Integration of activity in the higher plant. University Press, pp 471–505
Cowan IR, Troughton J (1971) The relative role of stomata in transpiration and assimilation. Planta 97:325–336
Cregg B (2004) Improving drought tolerance of trees: theoretical and practical considerations. In: Acta Horticulturae Evaluation, Production and Use, XXVI International Horticultural Congress: Nursery Crops; Development. Aug 11-17, 2002, pp 147–158
Dachnowski A (1914) Transpiration in relation to growth and to the successional and geographical distribution of plants. Ohio Nat 14:241–251
Daubeny C (1836) On the action of light upon the atmosphere. Philos Trans R Soc 126:149–175
De La Torre A, Puiu D, Langley CH et al (2019) Genomic architecture of complex traits in loblolly pine. New Phytol 221:1789–1801
de Saussure N (1804) Chemische Untersuchungen über die Vegetation. Leipzig, 1890
Dehérain M (1869) L’évaporation de l’eau et la decomposition de l’acide carbonique par les feuilles des végétaux. Aannales des Sci Nat–Bot 5(XVII):5–23
Dekker SC, Groenendijk M, Booth BBB, Huntingford C, Cox PM (2016) Spatial and temporal variations in plant water-use efficiency inferred from tree-ring, eddy covariance and atmospheric observations. Earth Syst Dyn 7:525–533
Dietrich T (1872) Ueber die durch unsere Culturpflanzen verdunsteten Wassermengen. Mitth des landw Cent für den Regierungsbezirk Cassel 1872:343
Dreibelbis F, Harrold L (1958) Water-use efficiency of corn, wheat, and meadow crops. Agron J 50:500–5003
Eckert AJ, Maloney PE, Vogler DR, Jensen CE, Mix AD, Neale DB (2015) Local adaptation at fine spatial scales: an example from sugar pine ( Pinus lambertiana , Pinaceae ). Tree Genet Genomes 11:42
Egerton FN (2001a) A history of the ecological sciences: early Greek origins. Bull Ecol Soc Am 82:93–97
Egerton FN (2001b) A history of the ecological sciences, part 4: Roman natural history. Bull Ecol Soc Am 82:243–246
Egerton FN (2002) A history of the ecological sciences, part 7: Arabic language science: botany, geography, and decline. Bull Ecol Soc Am 83:261–266
Egerton FN (2003) A history of the ecological sciences, part 10: botany during the Italian Renaissance and beginnings of the scientific revolution. Bull Ecol Soc Am 84:130–137
Egerton FN (2004a) A history of the ecological sciences, part 12: invertebrate zoology and parasitology during the 1500s. Bull Ecol Soc Am 85:27–31
Egerton FN (2004b) A history of the ecological sciences, part 13: broadening science in Italy and England, 1600–1650. Bull Ecol Soc Am 85:110–119
Egerton FN (2004c) A history of the ecological sciences, part 14: plant growth studies in the 1600s. Bull Ecol Soc Am 85:208–213
Egerton FN (2005) A history of the ecological sciences, part 16: Robert Hooke and the Royal Society of London. Bull Ecol Soc Am 86:93–101
Egerton FN (2008) A history of the ecological sciences, part 28: plant growth studies during the 1700s. Bull Ecol Soc Am 89:159–175
Egle K, Ernst A (1949) Die Verwendung des Ultrarotabsorptionsschreibers für die vollautomatische und fortlaufende CO 2 -Analyse bei Assimilations-und Atmungsmessungen an Pflanzen. Zeitschrift für Naturforsch B 4:351–360
Ehleringer J, White J, Johnson D, Brick M (1990) Carbon isotope discrimination, photosynthetic gas exchange, and transpiration efficiency in beans and range grasses. Acta Oecol 11:611–625
Farquhar G (1980) Carbon isotope discrimination by plants: effects of carbon dioxide concentration and temperature via the ratio of intercellular and atmospheric CO 2 concentrations. In: Carbon dioxide and climate: Australian research. Australian Academy of Science, Canberra, pp 105–110
Farquhar GD, Rashke K (1978) On the resistance to transpiration of the sites of evaporation within the leaf. Plant Physiol 61:1000–1005
Article CAS PubMed PubMed Central Google Scholar
Farquhar GD, Richards PA (1984) Isotopic composition of plant carbon correlates with water-use efficiency of wheat genotypes. Aust J Plant Physiol 11:539–552
Farquhar GD, O’Leary MH, Berry JA (1982) On the relationship between carbon isotope discrimination and the intercellular CO2-concentration in leaves. Aust J Plant Physiol 9:121–137
Farsky F (1877) Ueber die Wasserverdunstung von Korn, Gerste und Erbse. Chem List [Chemische Blätter] tom I
Fittbogen J (1871) Altes und Neues aus dem Leben der Gerstenpflanze. Landwirtsch Versuchs-Stationen 13:81–136
Gaastra P (1959) Photosynthesis of crop plants as influenced by light, carbon dioxide, temperature, and stomatal diffusion resistance. Meded van Landbouwhoogeschool Wageningen 59:1–68
Govindjee, Krogmann D (2004) Discoveries in oxygenic photosynthesis (1727-2003): a perspective. Photosynth Res 80:15–57
Grew N (1682) The anatomy of plants. W. Rawlins, London
Grinsted M (1977) A study of the relationships between climate and stable isotope ratios in tree rings. University of Waikato PhD Thesis
Hales S (1727) Vegetable staticks, or, an account of some statical experiments on the sap in vegetables : being an essay towards a natural history of vegetation : also, a specimen of an attempt to analyse the air, by a great variety of chymio-statical experiments. W. and J Innys and T Woodward, London
Hellriegel (1871) Wie viel Wasser beanspruchen unsere Getreidearten zur Production einer vollen Ernte? Amtliches Vereinsblatt des landwirtlischaftlichen Prov fuer die Mark Brand und Niederlausitz 3:60–62
Hellriegel H (1883) Beiträge zu den Naturwiss. Grundlagen des Ackerbaus. F, Vieweg und Sohn, Braunschweig
Hershey D (2003) Misconceptions about Helmont’s willow experiment. Plant Sci Bull 49:78–83
Hobart C, Harris K (1946) Fitting cropping systems to water supplies in Central Arizona. College of Agriculture, University of Arizona, Tucson, AZ, USA
Hopkins J (1996) Nicholas of Cusa on wisdom and knowledge. Arthur Banning Press, Minneapolis
Hubick K, Farquhar G, Shorter R (1986) Correlation between water-use efficiency and carbon isotope discrimination in diverse peanut ( Arachis ) germplasm. Aust J Plant Physiol 13:803–816
Iljin V (1916) Relation of transpiration to assimilation in steppe plants. J Ecol 4:65–82
Ingen-Housz J (1779) Experiments upon vegetables, discovering their great power of purifying the common air in the sunshine and of injuring it in the shade and at night. P. Elmsly, and H. Payne, London
Kearney TH, Shantz HL (1911) The water economy of dry-land crops. Yearb United States Dep Agric 10:351–362
Kiesselbach T (1916) Transpiration as a factor in crop production. Bull Agric Exp Stn Nebraska 6:19–38
King F (1889) Soil physics. Annu Rep Agric Exp Stn Univ Wisconsin 6:189–206
Kolkunov W (1905) Contributions to the problem of breeding drought resistant crop plants. I. Anatomical and Physiological investigations of the degree of xerophily of certain cereals. Mém Polytech Inst Kiev 5(4):
Lavoisier A-L (1770) Sur la nature de l’eau et sur les expériences par lesquelles on a prétendu prouver la possibilité de son changement en terre. Mémoires l’Académie des Sci:73–82
Lawes JB (1850) Experimental investigation into the amount of water given. J Hortic Soc London 5:38–63
Lawes JB (1851) Report upon some experiments undertaken at the suggestion of Professor Lindley, to ascertain the comparative evaporating properties of evergreen and deciduous trees. J Hortic Soc London 6:227–242
Lazenby EM (1995) The historia plantarum generalis of John Ray: book i - a translation and commentary. Newcastle University PhD thesis
Leather JW (1910) Water requirements of crops in India. Mem Dep Agric India Chem Ser 1(3):133–154
Leather JW (1911) Water requirements of crops in India. -II. Mem Dep Agric India Chem Ser 1:205–281
Lévesque M, Siegwolf R, Saurer M, Eilmann B, Rigling A (2014) Increased water-use efficiency does not lead to enhanced tree growth under xeric and mesic conditions. New Phytol 203:94–109
Linares J, Camarero J (2012) From pattern to process: linking intrinsic water-use efficiency to drought-induced forest decline. Glob Chang Biol 18:1000–1015
Luft K (1943) Über eine neue Methode der registrierenden Gasanalyse mit Hilfe der Absorption ultraroter Strahlen ohne spektrale Zerlegung. Z Tech Phys 24:97–104
Malpighi M (1675) Anatome Plantarum. Johannis Martyn, London
Marié-Davy H (1869) Evaporation du sol et des plantes. J d’Agriculture Prat 2:234–239
Marié-Davy H (1874) Note sur la quantité d’eau consommée par le froment pendant sa croissance. Comptes rendus Hebd des séances l’Académie des Sci 79:208–212
Maskell EJ, Blackman FF (1928) Experimental researches on vegetable assimilation and respiration. XVIII.—The relation between stomatal opening and assimilation.—A critical study of assimilation rates and porometer rates in leaves of Cherry Laurel. Proc R Soc Lond Ser B 102:488–533
Maximov NA (1929) The plant in relation to water. George Allen & Unwin LTD, London
Maximov NA, Alexandrov V (1917) The water requirement and drought resistance of plants. Trav du Jard Bot Tiflis 19:139–194
McCarroll D, Loader NJ (2004) Stable isotopes in tree rings. Quat Sci Rev 23:771–801
Medlyn BE, De Kauwe MG, Lin YS et al (2017) How do leaf and ecosystem measures of water-use efficiency compare? New Phytol 216:758–770
Meinzer FC, Ingamells JL, Crisosto C (1991) Carbon isotope discrimination correlates with bean yield of diverse coffee seedling populations. Hort Sci 26:1413–1414
Möbius M (1901) Die Anatomie der Pflanzen I: and II. Theil. Engelmann, W, Leipzig
Mohl H (1856) Welche Ursachen bewirken die Erweiterung und Verengung der Spaltöffnungen? Bot Zeitung 14:697–704
Möldenhawer J (1812) Beyträge zur Anatomie der Pflanzen. CL Wäser, Kiel
Montgomery E (1911) Methods of determining the water requirements of crops. Proc Am Soc Agron 3:261–283
Moore B (1924) Reviewed work: Physiological requirements of Rocky Mountain trees by Carlos G. Bates Ecology 5:298–302
Morton A (1981) History of botanical science: an account of the development of botany from ancient times to the present day. Academic Press, London
Nickelsen K (2007) From leaves to molecules: botany and the development of photosynthesis research. Ann Hist Philos Biol 12:1–40
Park R, Epstein S (1960) Carbon isotope fractionation during photosynthesis. Geochim Cosmochim Acta 21:110–126
Pellizzari E, Camarero JJ, Gazol A, Sangüesa-Barreda G, Carrer M (2016) Wood anatomy and carbon-isotope discrimination support long-term hydraulic deterioration as a major cause of drought-induced dieback. Glob Chang Biol 22:2125–2137
Penman HT, Schofield RK (1951) Some physical aspects of assimilation and transpiration. Symp Soc Exp Biol 5:115–129
Perrault C (1680) Essais de Physique. Jean Baptiste Coignard, Paris
Picon C, Guehl J-M, Aussenac G (1996) Growth dynamics, transpiration and water-use efficiency in Quercus robur plants submitted to elevated CO 2 and drought. Ann des Sci For 53:431–446
Plomion C, Bartholomé J, Bouffier L et al (2016) Understanding the genetic bases of adaptation to soil water deficit in trees through the examination of water use efficiency and cavitation resistance: maritime pine as a case study. J Plant Hyd 3:008
Polster H, Weise G, Neuwirth G (1960) Ecological researches on the CO 2 balance [net assimilation] and water economy of some tree species in sand and alkali soils in Hungary. Arch für Forstwes 9:947–1014
Porter R (1979) John Woodward; ‘A droll sort of philosopher’. Geol Mag 116:335–343
Priestley J (1775) Experiments and observations on different kinds of air. J.Johnson, London
Raber O (1937) Water utilization by trees, with special reference to the economic forest species of the north temperate zone. USDA Misc Pub No 257, Washington DC
Ray J (1686) Historia Plantarum, I edn. The Royal Society, London
Ray J (1691) The wisdom of God manifested in the works of creation ; first published in 1691: reprinted by the Wernerian Club, London 1844-1846
Roeser J (1940) The water requirement of Rocky Mountain conifers. J For 38:24–26
Rötzer T, Liao Y, Goergen K, Schüler G, Pretzsch H (2013) Modelling the impact of climate change on the productivity and water-use efficiency of a central European beech forest. Clim Res 58:81–95
Roupsard O, Joly HI, Dreyer E (1998) Variability of initial growth, water-use efficiency and carbon isotope discrimination in seedlings of Faidherbia albida (Del.) A. Chev., a multipurpose tree of semi-arid Africa. provenance and drought effects. Ann des Sci For 55:329–348
Rundel P, Ehleringer J, Nagy K (1989) Stable isotopes in ecological research. Springer-Verlag, New York
Book Google Scholar
Rüsch J (1959) Das Verhältnis von Transpiration und Assimilation als physiologische Kenngröße, untersucht an Pappelklonen. Theor Appl Genet 29:348–354
Scarth GW (1927) Stomatal movement: its regulation and regulatory role - a review. Protoplasma 2:498–511
Schmidt H-L, Winkler F (1979) Einige Ursachen der Variationsbreite von ẟ 13 C-Werten bei C3- und C4-Pflanzen. Ber Dtsch Bot Ges 92:S 185–S 191
Schröder M (1895) The transpiration of various crop plants. Agric For 10:320–336
Shantz HL (1927) Drought resistance and soil moisture. Ecology 8:145–157
Sharrock R (1660) The history of the propagation & improvement of vegetables. A. Lichfield, Oxford
Sinclair TR, Tanner CB, Bennett JM (1984) Water-use efficiency crop production. Bioscience 34:36–40
Sorauer P (1880) Studien über Verdunstung. Wollny - Forschungen auf dem Gebiete der Agrik tom 3:351–490
Spedding J, Ellis R, Heath D (1900) The works of Francis Bacon, Houghton, Mifflin and Company
Stanhill G (1986) John Woodward - a neglected 17th century pioneer of experimental botany. Isr J Bot 35:225–231
Tallec T, Béziat P, Jarosz N, Rivalland V, Ceschia E (2013) Crops’ water use efficiencies in temperate climate: comparison of stand, ecosystem and agronomical approaches. Agric For Meteorol 168:69–81
Tanner CB, Sinclair TR (1983) Efficient water use in crop production: research or re-search? In: Taylor HM, Jordan WR, Sinclair TR (eds) Limitations to efficient water use crop production. American Society of Agronomy, Madison, pp 1–27
Thornthwaite C (1947) Climate and moisture conservation. Ann Assoc Am Geogr 37:87–100
Tomic S, Cussenot M, Dreyer E (2005) La lumiére et les plantes : histoire de la découverte de la « photosynthése », 1779-1804. In: Changeux J-P (ed) La lumière au siècle des lumières et aujourd’hui: Art et science. Odile Jacob, Paris, pp 145–161
Troughton J (1969) Plant water status and carbon dioxide exchange of cotton leaves. Aust J Biol Sci 22:289–302
Tschaplowitz (1878) Ueber die Verdunstung und Substanzzunahme der Pflanzen. Berichte der Sect für Landwirtsch Versuchswes auf der Naturforscherversammlung zu München 1877(tome XX):74
Unger F (1862) Neue Untersuchungen über die Transpiration der Pflanzen. Sitzungsberichte der Kais Akad der Wissenschaften Wien 44:181–217 and 327-368
Vadez V, Kholova J, Medina S, Kakkera A, Anderberg H (2014) Transpiration efficiency: new insights into an old story. J Exp Bot 65:6141–6153
van Helmont J (1662) Oriatrike or Physick Refined. Lodowick Loyyd, London
Vialet-Chabrand S, Matthews JSA, Brendel O, Blatt MR, Wang Y, Hills A, Griffiths H, Rogers S, Lawson T (2016) Modelling water use efficiency in a dynamic environment: an example using Arabidopsis thaliana. Plant Sci 251:65–74
Viets FG (1962) Fertilizers and the efficient use of water. Adv Agron 14:223–264
Virgona J, Hubick K, Rawson H et al (1990) Genotypic variation in transpiration efficiency, carbon-isotype discrimination and carbon allocation during early growth in sunflower. Aust J Plant Physiol 17:207–214
Vivas M, Rolo V, Wingfield MJ, Slippers B (2019) Maternal environment regulates morphological and physiological traits in Eucalyptus grandis . For Ecol Man 432:631–636
Vogel JC (1980) Fractionation of the carbon isotopes during photosynthesis. In: Sitzungsberichte der Heidelberger Akademie der Wissenschaften. Springer, New York, pp 111–135
von Höhnel FR (1879) Ueber die Wasserverbrauchsmengen unserer Forstbäume mit Beziehung auf die forstlich-meteorologischen Verhältnisse. Wollny - Forschungen aus dem Gebiet der Agric tom II:398–421
von Schleiden MJ (1849) Grundzüge der wissenschaftlichen Botanik, 3rd edn. Verlag von Wilhelm Engelmann, Leipzig
von Seelhorst C (1902) Vegetationskästen zum Studium des Wasserhaushaltes im Boden. J Landwirtsch 50:277–280
Woodward J (1699) Some thoughts and experiments concerning vegetation. Philos Trans R Soc Lond A 21:193–227
Zhang J, Marshall JD (1994) Population differences in water-use efficiency of well-watered and water-stressed western larch seedlings. Can J For Res 24:92–99
Download references
Acknowledgements
Much of the historical background is based on A.G. Morton’s “History of Botanical Sciences” as well as to Frank N. Egerton’s “A History of the Ecological Sciences” series in the “Bulletin of the Ecological Society of America”. The author is also largely indebted to C. Schuchardt from the Library of the Staatsbetrieb Sachsenforst for help with the quest for rare German publications. The author would also like to thank E. Dreyer and J.M. Guehl (both from the SILVA Unit at INRAE Nancy, France) who commented extensively on an earlier version of the draft and J. Williams (University of Sussex), L. Handley and J. Raven (University of Dundee) who made many valuable suggestions and improved language.
Author information
Authors and affiliations.
Université de Lorraine, AgroParisTech, INRAE, UMR Silva, Nancy, France
Oliver Brendel
You can also search for this author in PubMed Google Scholar
Corresponding author
Correspondence to Oliver Brendel .
Ethics declarations
Conflict of interest.
The author declares no competing interests.
Additional information
Handling Editor: Andrew Merchant
Publisher’s note
Springer Nature remains neutral with regard to jurisdictional claims in published maps and institutional affiliations.
The original online version of this article was revised due to a retrospective Open Access order.
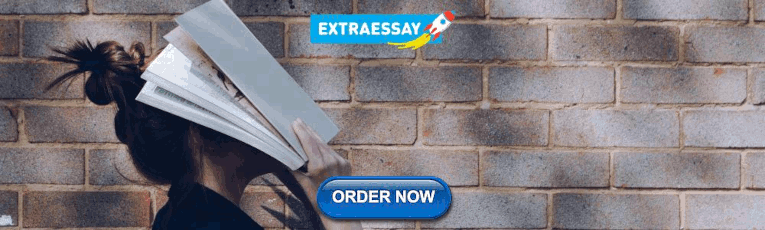
Rights and permissions
Open Access This article is licensed under a Creative Commons Attribution 4.0 International License, which permits use, sharing, adaptation, distribution and reproduction in any medium or format, as long as you give appropriate credit to the original author(s) and the source, provide a link to the Creative Commons licence, and indicate if changes were made. The images or other third party material in this article are included in the article's Creative Commons licence, unless indicated otherwise in a credit line to the material. If material is not included in the article's Creative Commons licence and your intended use is not permitted by statutory regulation or exceeds the permitted use, you will need to obtain permission directly from the copyright holder. To view a copy of this licence, visit http://creativecommons.org/licenses/by/4.0/ .
Reprints and permissions
About this article
Cite this article.
Brendel, O. The relationship between plant growth and water consumption: a history from the classical four elements to modern stable isotopes. Annals of Forest Science 78 , 47 (2021). https://doi.org/10.1007/s13595-021-01063-2
Download citation
Received : 26 November 2020
Accepted : 14 April 2021
Published : 28 April 2021
DOI : https://doi.org/10.1007/s13595-021-01063-2
Share this article
Anyone you share the following link with will be able to read this content:
Sorry, a shareable link is not currently available for this article.
Provided by the Springer Nature SharedIt content-sharing initiative
- Transpiration efficiency
- Water use efficiency
- Plant ecophysiology
- Botanical history
Annals of Forest Science
ISSN: 1297-966X
- Submission enquiries: [email protected]
- General enquiries: [email protected]
- Reference Manager
- Simple TEXT file
People also looked at
Original research article, the role of knowledge about water consumption in the context of intentions to use iot water metrics.
- Department of Commerce and Marketing, Institute of Marketing, Poznan University of Economics, Poznań, Poland
Technological progress can contribute to a more conscious and sustainable consumption of water. This is especially important in the context of dwindling resources and climate change. The objective of the study is to investigate how consumers’ perceived knowledge on water usage influences their intention to adopt smart (IoT-based) water meters, which deliver very precise data on the amount of water used in a household. We hypothesized that perceived knowledge on personal water usage exerts a direct and indirect influence on the intention to adopt a smart water meter. For the mediators, we used the intention to save water and variables derived from Value-Belief-Norm theory: awareness of consequences, ascription of responsibility and personal norm. We verified the hypotheses by applying structural equation modelling to a sample of 532 respondents. We found that perceived knowledge on water usage directly influences the intention to adopt a smart water meter, and that all considered variables worked to mediate the relationship between perceived knowledge and intention. Thus, based on our results perceived knowledge plays an important role on the relationship between values-beliefs-norms and intentions to apply smart water meters, which can be used for future research in order to reduce household water consumption by increasing the probability of installing smart water meters.
Introduction
Water is a fundamental resource to human life, integral to our personal survival and to everything that societies produce ( Cosgrove and Loucks, 2015 ). Water is a finite resource that has no substitutes—and yet, there is growing proof that human activities are contributing to water scarcity across the globe ( Fielding et al., 2012 ). Such activities include the expansion of businesses, urbanization, population growth, water pollution, increasing water demands, the overallocation of rivers flows, and climate change ( Cosgrove and Loucks, 2015 ). The consequences of water scarcity are far-reaching, encompassing food insecurity, the destruction of eco-systems, the extinction of species and social stress ( Addo et al., 2019 ). Past research has found that about one fifth of the world population lives in areas that lack sufficient water to meet all demands, while one third of the population does not have access to clean drinking water ( Cosgrove and Loucks, 2015 ). As population growth spurs greater water usage, more and more parts of the world will face water stress.
One of the leading causes of water scarcity is increasing domestic water use—the demand for which is primarily driven by high consumption in urban centers, households and industrial sectors. In fact, domestic demand is said to comprise about 15% of the global water demand ( Addo et al., 2019 ). Thus, governments, policymakers, and citizens are challenged to find ways to reduce household water consumption while satisfying the water demands of society at large ( Lowe et al., 2015 ). In households, about half of water usage is dedicated to indoor activities, including toilets, showers, washing machines and other daily activities ( Shan et al., 2015 ) and the other half of water consumption is dedicated to outdoor activities ( Lee et al., 2011 ). According to past research, the factors that influence household water consumption are number of people in a household, education level, infrastructure, income, devices, usage patterns, lifestyle and consumer attitudes toward water consumption ( Willis et al., 2011 ; Rondinel-Oviedo and Sarmiento-Pastor, 2020 ). Regardless of the reason, societies need to implement conservation practices in order to achieve sustainable water usage. Lee et al. (2011) advanced that applying water conservation as the residential level is important since households account for the majority of water demand in cities.
Despite the need to reduce household water consumption, there is a lack of research on the best ways to do so. This is primarily due to the complexity behind the drivers of water-saving behavior. According to Jorgensen et al. (2009) , there are two groups of behavioral drivers: direct (climate variability, financial incentives and disincentives, regulations, property and household characteristics) and indirect (socio-economic factors, inter-personal factors and institutional trust). Both play an important role in water-saving behavior. We want to highlight that personal characteristics occupy both spaces: the intention to and knowledge of how to conserve water was found to be a direct driver, whereas environmental values and conservation attitudes are recognized as an indirect one.
Based on past literature review, the research area about household water conservation has been focused on consumers’ demographics and characteristics, pricing, the efficient use of household appliances, and consumer-focused messaging ( Madias and Szymkowiak 2022 ). Fielding et al. (2012) discovered that demographic variables are among the strongest predicts of conservation behaviors. Cary (2008) analyzed the ways to influence consumers’ behavior and attitudes in order to reduce their water consumption. Similarly, Corral-Verdugo et al. (2003) found that consumers who are more concerned about the environment are more likely to adjust their water habits, such as limiting their time taking showers, washing dishes, watering plants, etc. Scholars have also established that water pricing plays an important role in consumers’ conservation practices ( Dupont and Renzetti, 2013 ). Likewise, residents will sometimes modify their water consumption in response to conservation messages ( Addo et al., 2019 ).
Moving beyond consumers, research has examined methods of making household appliances more environmentally efficient ( Belke et al., 2019 ; Boyano and Moons, 2020 ; Pakula and Stamminger, 2010 ) in order to counteract households’ relatively high water usage. One of the way to achieve that is through Green Technology, which refers to technology that helps to achieve the international sustainable development goals and to minimize the environmental impact cause by economic growth ( Ikram et al., 2022 ). Smart water meters are also considered as green technology as it is proven that they play an important role on reducing the household water consumption ( Russell and Knoeri, 2020 ) as they can track people’s real-time water consumption and detect any water possible leakage ( Fuentes and Mauricio 2020 ). In fact, Sønderlund et al. (2014) found that households reduced their water consumption by an average of 19.6% when they received information from a smart water meter. Cominola et al. (2021) did not replicate the size of this effect, they still found an 8% reduction in water consumption among households with smart meters installed.
However, past research has not examined consumers’ intentions to adopt smart water meters in their households which could help with understanding better the water consumption behavior but they mostly focus on the financial motives behind applying smart water meters ( Montginoul and Vestier 2018 ). Thus, this paper focuses on how consumers’ perceived knowledge about water usage shapes their intention to install smart (IoT-based) water meters. In this way, we address the scientific gap, as well as provide valuable insights for future research on smart water meters. We hypothesized that perceived knowledge (PK) on water usage in a household determines the intention to adopt smart water meters (IAW), both directly and indirectly. In this study, we followed other studies that treated water-saving as a pro-environmental behavior ( Carmela and Damiano, 2016 ), which is aligned with some theory on perceived knowledge, so we assumed that perceived knowledge is also related to intention to save water and to VBN variables ( Esfahani et al., 2015 ) which are usually adopted for explaining pro-environmental behavior. We also examined how consumers’ perceived knowledge about household water usage is related to their personal norm about saving water, and whether that norm results from their awareness of the consequences and ascription of responsibility in relation to excess water usage.
This paper is structured as follows: The first section reviews the relevant literature in order to synthesize existing knowledge and construct our hypotheses. The second section details our methodology. The third section presents our results, while the fourth discusses and interprets them. The final section summarizes our main contributions and outlines future research directions.
Hypotheses development
In order to construct our hypotheses we adopted the Value-Belief-Norm model by Stern et al. (1999) and extended it by adding the moderator of perceived knowledge. VBN model is primary used for examining green behaviors by researching individuals’ values, beliefs and norms ( Ghazali et al., 2019 ). The variables that the model is using in order to investigate the pro-environmental intentions of consumers are: ascription of responsibility, awareness of consequences and personal norms. However, in this study we extend the VBN model by adding the moderator or perceived knowledge. Thus, the hypotheses are constructed based on an extended version of VBN model.
Our primary focus is on consumers’ perceived knowledge and its influence (both direct and indirect) on their intention to adopt smart water meters. Past research has established that consumers’ knowledge and perceived knowledge are key factors in their decision-making, especially in relation to the environment ( Lee et al., 2006 ). Indeed, knowledge in general determines behavioral intentions ( Martono et al., 2019 ), while environmental knowledge in particular is related to pro-environmental behavior ( Levine and Strube, 2012 ; Suryanda et al., 2021 ). According to Min-Seong Kim et al. (2018) , environmental knowledge can be categorized into two parts: real knowledge and perceived knowledge (i.e., someone’s feeling of knowing something). Here, we focus on the latter category in relation to water consumption.
Perceived knowledge on water usage reflects people’s opinions on two issues: their perception of absolute water consumption and how much they use relative to the average person. Previous studies have highlighted a discrepancy between perceived and actual water consumption ( Beal et al., 2013 ). However, we assume that consumers who are more confident in their perceived knowledge (regardless of its accuracy) will be more interested in possessing precise data about their water usage—and thus will be more willing to adopt smart water meters. To that end, we formulated our first hypothesis:
H1. Perceived knowledge on personal water usage is positively related to the intention to adopt smart water meters.We know from past research that perceived knowledge can compel consumers to make environmentally friendly decisions ( House et al., 2004 ; Kim et al., 2018 ). From this, we postulate that consumers with higher perceived knowledge on water usage will have higher intentions to save water:
H2. Perceived knowledge on personal water usage is positively related to the intention to save water.Past research focusing on water meters have proved that smart-water meters may reduce the household water consumption on an average rate of 19.6% ( Sønderlund et al., 2014 ) while Davies et al. (2014) found out a reduction of 1.05 kl per month in water consumption when smart water meters were applied. Thus, we assume that consumers who have higher intentions to reduce their water consumption are more likely to have high intentions of adopting smart water meters. Formally:
H3. The intention to save water is positively related to the intention to adopt smart water meters.In numerous previous studies, pro-environmental behaviors and behavioral intentions have been explained by personal norm ( Schwartz, 1977 ; De Groot and Steg, 2009 ; Jansson et al., 2017 ): an internalized behavioral standard ( Bamberg, 2012 ) that is contrasted with subjective norms. Personal norm is a key construct in two theories used to explain pro-environmental behavior. Schwartz’s (1977) Norm Activation Theory (NAT) focuses on moral (personal) norm as a main motivator of altruistic behaviors, including pro-environmental ones. The theory posits that individuals undertake pro-environmental actions due to a personal belief that environmental conditions pose a threat to other people and all other species (awareness of consequences) and those harmful consequences can be prevented by their actions (ascription of responsibility). According to NAT, these types of actions are often rewarded by a sense of pride, security, fulfilling one’s duty and experiencing joy as a result, as well as enhanced self-esteem. Meanwhile, Stern et al. (1999) Value-Belief-Norm (VBN) theory extends NAM to a broader context by establishing causal links between the following variables: values (especially altruistic ones); beliefs about the environment and the effects of human activity on it; an awareness of consequences; the ascription of responsibility; personal norms concerning pro-environmental behavior, the willingness to sacrifice, and consumer behavior. Both the intention to and action of saving water should be considered highly pro-environmental and pro-social behavior ( Sulaeman et al., 2018 ). Thus, we assume that pro-environmental personal norms about saving water influence the intention to reduce household water consumption. This leads to our fourth hypothesis:
H4. The personal norm about saving water is positively related to the intention to save water.Regarding the influence of perceived knowledge on pro-environmental behaviors, Bamberg (2012) concluded that perceived knowledge about environmental problems is highly predictive of people’s norms development personal Similarly, Onel and Mukherjee (2016) found that the former is a better predictor for the latter than actual scientific and environmental knowledge. Hamzah and Tanwir (2021) established that environmental knowledge (which can be defined as perceived knowledge based on how they measured the variable) is a moderator of perceived green value, green purchase attitude, perceived behavioral control, subjective norms and their impact on green purchase intention. From this, we hypothesize that perceived knowledge on environmental issues is related to the intention to save water:
H5. Perceived knowledge on water usage is positively related to the personal norm about saving water.According to both NAT ( Schwartz, 1977 ) and VBN ( Stern et al . , 1999 ), personal norm is predicted by both an awareness of environmental consequences and the ascription of responsibility ( Esfahani et al., 2015 ). The ascription of responsibility is defined as the feeling of responsibility for the negative consequences of failing to act pro-socially ( De Groot and Steg, 2009 ). It also reflects an opinion about who should be responsible for something ( Stern, 2000 ). According to VBN, this factor mainly arises from the awareness of consequences, but it can also be driven by other factors like one’s internal locus of control ( Pavalache-Ilie and Unianu, 2012 ). Given previous findings that knowledge and problem awareness influence pro-environmental attitudes and behaviours ( Bamberg and Möser, 2007 ), we formulated the following hypothesis:
H6. Perceived knowledge on water usage is positively related to the ascription of responsibility for excessive water usage.Based on both NAT and VBN ( Schwartz, 1977 ; Stern et al., 1999 ), we expect to find a positive relation between the ascription of responsibility for excessive water usage and the personal norm about saving water. This leads us to the next hypothesis:
H7. Ascription of responsibility for excessive water usage is positively related to the personal norm about saving water.The awareness of consequences is the belief that taking (or failing to take) a given action will be harmful for others ( De Groot and Steg, 2009 ; Kiatkawsin and Han, 2017 ). In this study, we assume that consumers’ perceived knowledge about water usage will influence their awareness of consequences. Formally:
H8. Perceived knowledge on water usage is positively related to the awareness of consequences about excessive water usage.Previous studies ( Vining and Ebreo, 2002 ; Shin et al., 2018 ) have argued that the awareness of consequences is an antecedent to ascription of responsibility, as individuals tend to feel responsible for negative consequences when they are aware of inflicted harm. In our study, we assume that individuals who are aware of the consequences of excessive water usage will take personal responsibility for said consequences. Formally:
H9. The awareness of consequences about excessive water usage is positively related to the ascription of responsibility for using too much water.Lastly, prior research has uncovered that consumers’ awareness of consequences for taking (or not taking) a certain activity determines their personal norm about a given behavior ( Liu et al., 2017 ). Thus, we hypothesized the following:
H10. The awareness of consequences of excessive water usage is positively related to the personal norm about saving water.We built the following research model ( Figure 1 ) to visualize our hypotheses.
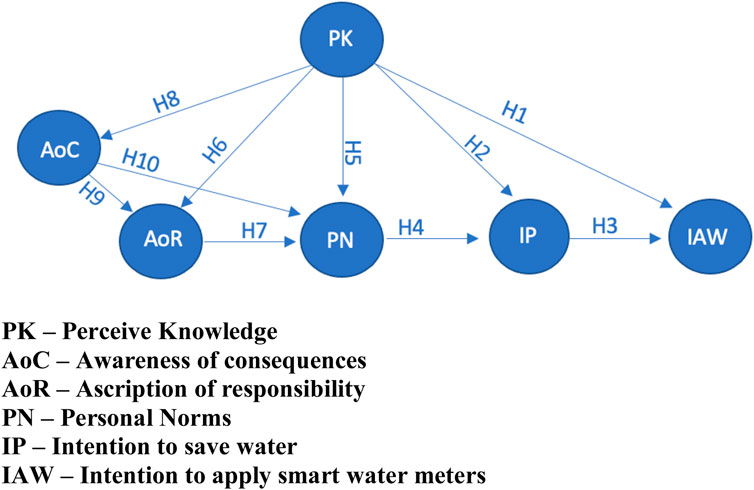
FIGURE 1 . Research model.
We designed a three-part study to empirically verify the above model. In the first part, participants received basic information about smart water meters. The description only contained technical information about the meter’s mechanical operation, without any emphasis on or reference to possible benefits. The description also showed example pictures of current devices on the market, which are targeted at individual customers, as well as a visualization of web and mobile applications, through which device users can access consumption information. This description was especially important for estate the credibility of the research, as it allowed users to obtain knowledge in the field of smart water metrics solutions. Moreover, it is related to the fact that such solutions are treated as a product and process innovation, so they are not widely known to all consumers.
In the second part, respondents used one to seven scales (where 1 = I strongly disagree and 7 = I strongly agree) to give their opinion on various statements. We derived the statements from previously validated scales: The study by Shin et al. (2018) was used to evaluate Ascription of responsibility for using too much water (AOR), which was measured on three items, as well as the Awareness of the consequences of using too much water (AOC) (e.g., I think that using too much water supports environmental degradations). In addition, personal norm concerning saving water (PN) was examined on three items derived by Shin et al. (2018) (e.g., “I believe I have a moral obligation to save water”). We measured Perceived Knowledge (PK) on personal water usage using a modified four-item scale proposed by Joshi and Rahman (2017) (e.g., “I am very knowledgeable about my water usage”). To evaluate the Intention to reduce water usage (IP) and the Intention to adopt IoT water meters (IAW), we adopted questions from Han et al. (2010) and Chen and Tung (2014) (e.g., “I will make an effort to reduce water usage”, “I plan to apply IoT based water meters in my household”) ( Appendix A1 ). In the final part, respondents answered questions about their demographics.
We conducted the survey over Amazon Mechanical Turk (hereafter, Mturk). Although MTurk data has proven to be of good quality, we still undertook a multistage verification procedure. We limited the participant pool to English-speaking United States residents, as well as those users with a high rate of completed tasks (over 90%). In addition, we eliminated anyone who completed the first part (reading the information about smart water metrics) in less than 15 s, as well as so-called “speed runners” who answered the main questions faster than 80% of the average pre-test time. Moreover, we implemented two attention check questions to verify careful reading and removed any respondents who failed them. In the last step, we excluded any participants who gave unlikely answers to open-ended questions (e.g., listing 1,111 years as their given age). Through this process, we achieved 532 responses for the core analysis. This final sample differed in terms of age (m = 40.1, SD-13.46, min. = 18, max. = 76), gender (male-44.55%, female-55,45%), household size (M = 3.0, SD = 1.39, min. = 1, max. = 9) income, education, status and type of residence. Table 1 presents the detailed respondent data.
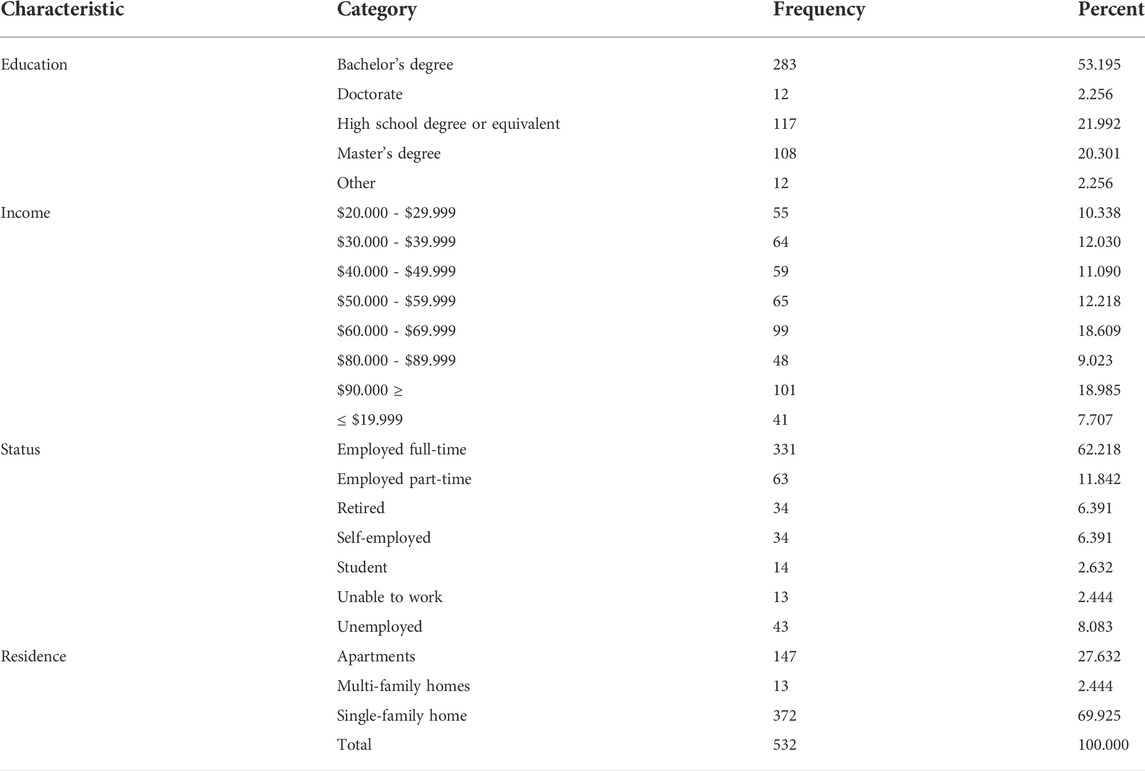
TABLE 1 . Respondent characteristics.
We analyzed the data in two stages using structural equation modeling. Following recommendations by Bagozzi and Yi (2012) , we first evaluated the convergent and discriminant validity of individual items, as well as the composite reliability of the variables. A confirmatory factor analysis indicated that all factor loadings for the individual items achieved values above 0.821, in excess of the recommended 0.6 threshold ( Chin et al., 1997 ). Next, we measured scale reliability by applying Cronbach’s α: The values ranged from 0.89 to 0.93, representing good to very good consistency according to Hair et al. (2014) . To measure convergent and discriminant validity, we used standardized factor loading along with two parameters: Composite Reliability (CR) and Average Variance Extracted (AVE). The AVE values were between 0.73 and 0.83 ( Table 2 ), which is above the acceptable limit of 0.5 as recommended by Hair et al. (2014) . The CR values also exceeded the acceptable limit of 0.6 (ranging from 0.89 to 0.93), which indicates the internal consistency of multiple indicators ( Bagozzi and Yi 2012 ). We used the HTMT ratio of correlations to assess the discriminant validity: As shown in Table 3 , the ratios of each construct were below 0.9, and thus established discriminant validity ( Henseler et al., 2014 ).
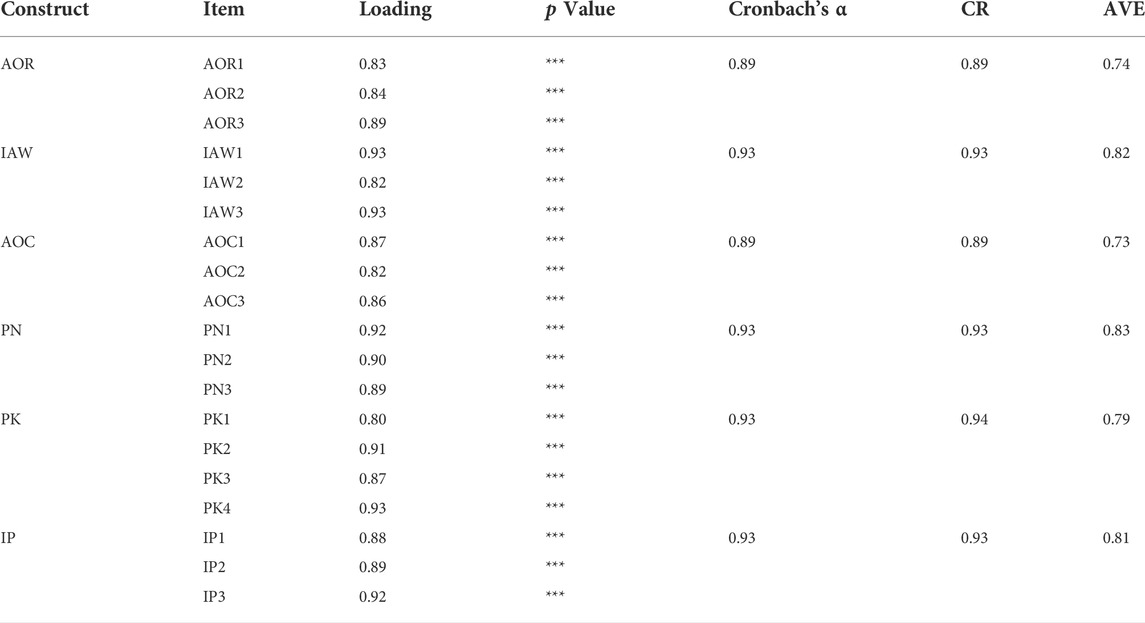
TABLE 2 . Confirmatory factor analysis.
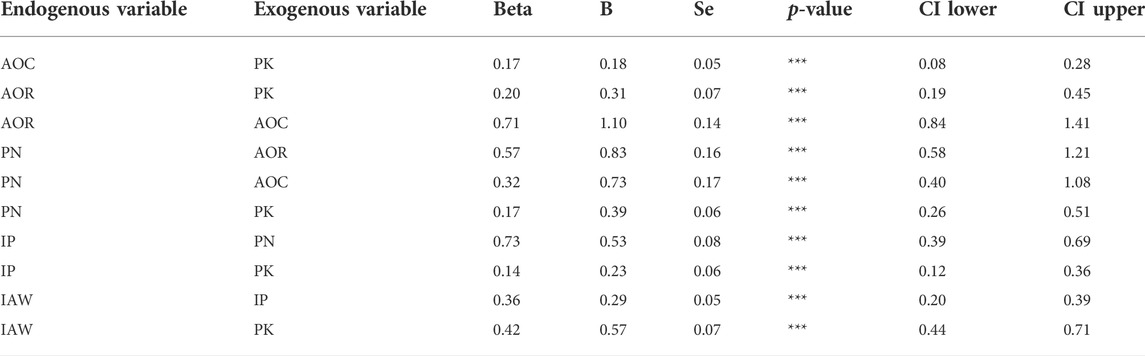
TABLE 3 . SEM analysis.
Following Schumacker and Lomax (1996) , we applied three types of fit indices to evaluate the model: absolute fit, parsimonious fit, and incremental fit. All the obtained fit indices met the suggested ranges like CMIN/df = 2.47, RMSEA = 0.538, GFI = 0.978, AGFI = 0.911, CFI = 0.964 and NFI = 0.911 ( Hair et al., 2014 ).
After confirming the reliability and validity of the measurement model, we performed path analysis to evaluate the relationships among the latent variables. We used the R programming environment and Lavaan, Psych package for this purpose, incorporating bootstrapping (2,000 re-samples) to improve the reliability of the results.
The applied structural model explains the high variability of the IP ( R 2 = 0.636), PN ( R 2 = 0.810) and IAW ( R 2 = 0.44) ( Table 4 ). In line with hypotheses H1 and H2 ( Figure 2 ), we confirmed that the PK of water consumption influenced both the intention to use smart water metrics (b = 0.42, p < 0.001) and the intention to reduce water (b = 0.14, p < 0.001). Additionally, the study confirmed hypothesis H3: The Intention to save water was positively related to the intention to adopt smart water meters (b = 0.36 p < 0.001). Further, we established the direct influence of all theoretical variables on Personal Norms, i.e., AOR (b = 0.57 p < 0.001), AOC (b = 0.32 p < 0.001), and PK (b = 0.17 p < 0.001). In line with hypothesis H4, the Personal Norm on saving water was positively related to the intention to save water (b = 0.73, p < 0.001). We also found that PK influenced AOR (b = 0.17 p < 0.001) and AOC (b = 0.20, p < 0.001), in respective alignment with H5 and H6. As we assumed, AOC had a strong direct effect on AOR (b = 0.71, p < 0.001) and on PN (b = 0.32, p < 0.001), in accordance with H8 and H9, respectively. Lastly, AOR seemed to influence PN, in support of H10 (b = 0.57, p < 0.001).
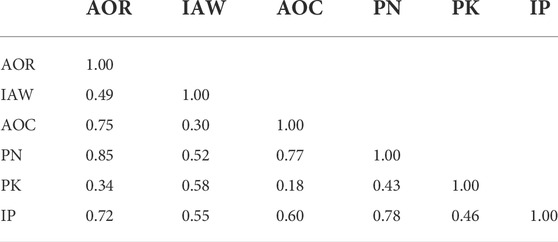
TABLE 4 . Discriminant validity (HTMT).
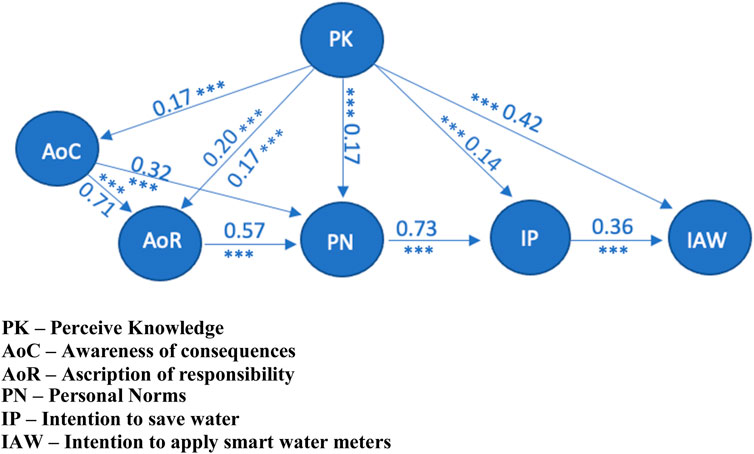
FIGURE 2 . SEM results.
This study found that consumers’ perceived knowledge about personal water usage has both a direct and indirect impact on their intentions to adopt smart water meters. The direct impact of perceived knowledge on intentions to apply smart water meters was indicated by an effect of strength 0.42. From this result, we assume that people who are more aware of their water consumption (which proxies their general interest and involvement in this issue) are also more willing to adopt smart meters that will provide more precise data.
We also uncovered that perceived knowledge indirectly influences the intention to save water. This corroborates prior research arguing that knowledge influences pro-environmental behaviors ( Levine and Strube, 2012 ; Suryanda et al., 2021 ). It follows that consumers who are willing to save water are also more eager to implement smart meters that can help them achieve that goal ( Sønderlund et al., 2014 ). In order to calculate the strength of the indirect effect, we multiplied the direct effects of intentions to save water towards intentions to apply smart water meters with the indirect effect of perceived knowledge to intentions to save water.
Notably, perceived knowledge exerted an indirect impact in several ways. The first was through personal norms. Personal norms are significantly connected with people’s perceived knowledge of personal water use and also impact the intention to reduce water (and by extension, adopt smart meters). Thus, our results affirm prior studies positing that personal norms can explain pro-environmental behaviors ( Schwartz, 1977 ; De Groot and Steg, 2009 ; Jansson et al., 2017 ).
In addition, perceived knowledge impacts the ascription of responsibility and the awareness of consequences, in support of the VBN theory. However, VBN argues that personal norms arise from both the awareness of consequences and ascription of responsibility. By contrast, we found that ascription of responsibility is individually, indirectly and significantly impacting personal norms. Thus, ascription of responsibility is impacting personal norms which influence the intention to reduce water consumption which impacts the intentions to apply smart water meters.
Furthermore, perceived knowledge of personal water use is also indirectly impacting the intentions to implement smart water meters through ascription of responsibility and awareness of consequences of excessive water use, we calculated the strength of this effect by multiplying the indirect and direct impacts. These variables are based on the Norm Activation model. Based on our founding knowledge is impacting both of these variables. Awareness of consequences requires some knowledge on the effects of water consumption in order to be determined, meanwhile ascription of responsibility is requiring awareness of consequences of excessive water consumption in order to be determined. Moreover, based on our results and the original model ascription of responsibility and awareness of consequences are impacting personal norms, which are influencing the intentions to reduce water which leads to higher intentions to implement smart water meters in order to reduce water consumption. Our results also serve to extend the VBN model. According to this theory, personal norms stem from the awareness of consequences and the ascription of responsibility. However, our research suggests that personal norms do not necessarily require this connection, and can instead be impacted by other variables, such as perceived knowledge.
Lastly, we want to highlight that the majority of past research has focused on people’s real environmental knowledge without considering their perceived knowledge ( Bang et al., 2000 ; Mostafa, 2006 ; William et al., 2009 ). For instance, Levine and Strube (2012) sought to determine whether environmental knowledge encourages environmentally friendly behavior; however, they adopted a questionnaire from NEETF that used questions such as “what is biodiversity” to measure real environmental knowledge. However, previous research suggests that green behaviors are associated more with perceived knowledge than objective knowledge ( House et al., 2004 ). Kim et al. (2018) confirmed that perceived knowledge is a strong predictor of environmental friendly behavior. Our results contribute to this latter stream by affirming that perceived knowledge can directly and indirectly influence the intention to engage in environmental behaviors, such as adopting smart water meters.
The main aim of this research paper was to broaden our understanding and extend the scientific knowledge about consumer behavior when it comes to pro-environmental decisions such as applying smart water meters in order to reduce their water consumption. Based on our results, we can say that perceived knowledge is an important variable that can directly and indirectly influence consumers’ intentions to adopt smart water meters in their households. In addition, we demonstrated that different variables impact consumers’ decision to implement smart water meters. Thus, our findings may hold value for future studies on implementing smart water meters at the household level. Contrary to traditional water meters, the use of IoT gives residents the insight to constantly and precisely measure the place, time and context of water use, including the possibility of determining the possibility of determining an individual residents’ water consumption. This knowledge can increase individual responsibility and possibly translate into a more efficient use of resources. Moreover, this research managed to verify that perceived knowledge of consumers in addition to their values-beliefs-norms can influence consumers to behave in a more sustainable manner.
In addition, based on our results, not only we managed to extend the scientific knowledge about consumers’ behavior towards water consumption but also to find ways in order to influence them to behave more sustainably by applying smart water meters and reducing their water consumption. The results could be used not only from researchers in order to extend the research area, but also from policy makers in order to create successful campaigns aiming on reducing household water consumption which plays an important role on the global water scarcity matter. IoT water meter manufactures could also use the results to not only make their product more appealing but also to contribute in water scarcity. The results present that there are ways to influence consumers to behave more sustainably and that could help with the global issue of water scarcity.
That said, our study features several limitations that may spur further research. First, we did not determine if any participants were living in water-stressed areas and how that might have influenced their answers. Second, most of our participants were well educated people, whereas as it was found out that socio-economic characteristics of consumers influenced their perceived water usage ( Beal et al., 2013 ), so as more of our responders are well educated that may impact our findings. We also did not examine the source of respondents’ perceived knowledge: We assumed that it was connected with people’s general interest in the quantity of water used, but it could also stem from a general belief in one’s knowledge superiority.
Regarding future research directions, scholars could explore other predictors of the intention to adopt smart water meters: for instance, by using theories of technology acceptance as the main theoretical framework, like the Technology Acceptance Model, the Unified Theory of Acceptance and Use of Technology (UTAUT2), or the Theory of Planned Behavior. Additionally, future research could investigate the influence of some moderating variables (e.g., environmental concern, frugality, or personal innovativeness) on the relationship between the perceived usefulness and ease of IoT water meters and the intention to adopt them. Lastly, while our research underscores the role of one’s willingness to save water in the decision to adopt smart water meters, future research could assess how various motives (e.g., financial, environmental) shape this relationship.
Data availability statement
The original contributions presented in the study are included in the article/Supplementary Material, further inquiries can be directed to the corresponding author.
Ethics statement
The studies involving human participants were reviewed and approved by The Committee of Ethical Science Research of Poznan University of Economics provided us with approval for the research. Written informed consent for participation was not required for this study in accordance with the national legislation and the institutional requirements.
Author contributions
KM and BB contributed to the conception and design of the study. AS organized the database. AS performed the statistical analysis. KM wrote the first draft of the manuscript. KM, BB, and AS wrote sections of the manuscript. BB and KM reviewed the manuscript. BB found funding for the research. All authors contributed to manuscript revision, read, and approved the submitted version.
The project is financed within the Regional Initiative for Excellence programme of the Minister of Science and Higher Education of Poland, years 2019-2022, grant no. 004/RID/2018/19, financing 3,000,000 PLN.
Conflict of interest
The authors declare that the research was conducted in the absence of any commercial or financial relationships that could be construed as a potential conflict of interest.
Publisher’s note
All claims expressed in this article are solely those of the authors and do not necessarily represent those of their affiliated organizations, or those of the publisher, the editors and the reviewers. Any product that may be evaluated in this article, or claim that may be made by its manufacturer, is not guaranteed or endorsed by the publisher.
Addo, I. B., Thoms, M. C., and Parsons, M. (2019). The influence of water-conservation messages on reducing household water use. Appl. Water Sci. 9 (5), 126. doi:10.1007/s13201-019-1002-0
CrossRef Full Text | Google Scholar
Bagozzi, R. P., and Yi, Y. (2012). Specification, evaluation, and interpretation of structural equation models. J. Acad. Mark. Sci. 40 (1), 8–34. doi:10.1007/s11747-011-0278-x
Bamberg, S. (2012). “Understanding and promoting bicycle use – insights from psychological research” in Cycling and Sustainability (Transport and Sustainability, Vol. 1) . Editors J. Parkin (Bingley: Emerald Group Publishing Ltd ), 219–246. doi:10.1108/S2044-9941(2012)0000001011
Bamberg, S., and Möser, G. (2007). Twenty Years after Hines, Hungerford, and Tomera: A New Meta-Analysis of Psycho-Social Determinants of Pro-Environmental Behavior. J. Environ. Psychol. 27, 14–25. doi:10.1016/j.jenvp.2006.12.002
Bang, H.-K., Ellinger, A. E., Hadjimarcou, J., and Traichal, P. A. (2000). Consumer concern, knowledge, belief, and attitude toward renewable energy: An application of the reasoned action theory. Psychology & Marketing 17, 449–468.
Beal, C. D., Stewart, R. A., and Fielding, K. (2013). A novel mixed method smart metering approach to reconciling differences between perceived and actual residential end use water consumption. J. Clean. Prod. 60, 116–128. doi:10.1016/j.jclepro.2011.09.007
Belke, L., Maitra, W., Nijhuis, B., Presti, C., and Stamminger, R. (2019). Global consumer study to identify water wastage in the usage of automatic dishwashers. Int. J. Consum. Stud. 43 (5), 471–479. doi:10.1111/ijcs.12526
Boyano, A., and Moons, H. (2020). Analysing of the impacts of the revision of Ecodesign and Energy Label Regulations for household dishwashers by using a stock model. Energy Effic. 13 (6), 1147–1162. doi:10.1007/s12053-020-09874-4
Carmela, A. M., and Damiano, F. (2016). Conservation behavior and environmental concerns . Naples, Italy: University of Napoli Parthenope, Italy. University of Napoli Parthenope , 75065.
Google Scholar
Cary, J. W. (2008). Influencing attitudes and changing consumers’ household water consumption behaviour. Water Sci. Technol. Water Supply 8 (3), 325–330. doi:10.2166/ws.2008.078
Chen, M.-F., and Tung, P.-J. (2014). Developing an extended Theory of Planned Behavior model to predict consumers’ intention to visit green hotels. Int. J. Hospitality Manag. 36, 221–230. doi:10.1016/j.ijhm.2013.09.006
Cominola, A., Giuliani, M., Castelletti, A., Fraternali, P., Herrera, S., Guardiola, J. C., et al. (2021). Long-term water conservation is fostered by smart meter-based feedback and digital user engagement. npj Clean Water 4 (1), 29. doi:10.1038/s41545-021-00119-0
Davies, K., Doolan, C., van den Honert, R., Shi, R., et al. (2014). Water-saving impacts of Smart Meter technology: An empirical 5 year, whole-of-community study in Sydney, Australia. Water Resour. Res. 50, 7348–7358. doi:10.1002/2014WR015812
Chin, W. W., Gopal, A., and Salisbury, W. D. (1997). Advancing the theory of adaptive structuration: The development of a scale to measure faithfulness of appropriation. Inf. Syst. Res. 8 (4), 342–367. doi:10.1287/isre.8.4.342
Corral-Verdugo, V., Bechtel, R. B., and Fraijo-Sing, B. (2003). Environmental beliefs and water conservation: An empirical study. J. Environ. Psychol. 23 (3), 247–257. doi:10.1016/S0272-4944(02)00086-5
Cosgrove, W. J., and Loucks, D. P. (2015). Cosgrove și loucks2015-Water_Resources_Research.pdf , 4823–4839. doi:10.1002/2014WR016869.Received
De Groot, J., and Steg, L. (2009). Morality and prosocial behavior: The role of awareness, responsibility, and norms in the norm activation model. J. Soc. Psychol. 149 (4), 425–449. doi:10.3200/SOCP.149.4.425-449
PubMed Abstract | CrossRef Full Text | Google Scholar
Dupont, D. P., and Renzetti, S. (2013). Household behavior related to water conservation. Water Resour. Econ. 4, 22–37. doi:10.1016/j.wre.2013.12.003
Esfahani, M. D., Nilashi, M., Rahman, A. A., Ghapanchi, A. H., and Zakaria, N. H. (2015). Psychological factors influencing the managers’ intention to adopt green IS. Int. J. Strategic Decis. Sci. 6 (2), 28–56. doi:10.4018/ijsds.2015040103
Fielding, K. S., Russell, S., Spinks, A., and Mankad, A. (2012). Determinants of household water conservation: The role of demographic, infrastructure, behavior, and psychosocial variables. Water Resour. Res. 48 (10), 2012WR012398. doi:10.1029/2012WR012398
Fuentes, H., and Mauricio, D. (2020). Smart water consumption measurement system for houses using IoT and cloud computing. Environ. Monit. Assess. 192 (9), 602. doi:10.1007/s10661-020-08535-4
Ghazali, E. M., Nguyen, B., Mutum, D. S., and Yap, S.-F. (2019). Pro-environmental behaviours and value-belief-norm theory: Assessing unobserved heterogeneity of two ethnic groups. Sustainability 11 (12), 3237. doi:10.3390/su11123237
Hair, J., Black, W., Babin, B., and Anderson, R. (2014). Multivariate data analysis . 7th Edition. Essex: Pearson Education Limited .
Han, H., Hsu, L., and Sheu, C. (2010). Application of the Theory of Planned Behaviour to Green Hotel Choice: Testing the Effect of Environmentally Friendly Activities. Tourism Manag. 31 (3), 325–334. doi:10.1016/j.tourman.2009.03.013
Hamzah, M. I., and Tanwir, N. S. (2021). Do pro-environmental factors lead to purchase intention of hybrid vehicles? The moderating effects of environmental knowledge. J. Cleaner Prod. 279, 123643. doi:10.1016/j.jclepro.2020.123643
Henseler, J., Ringle, C. M., and Sarstedt, M. (2014). A new criterion for assessing discriminant validity in variance-based structural equation modeling. J. Acad. Mark. Sci. 43 (1), 115–135. doi:10.1007/s11747-014-0403-8
House, L., Lusk, J., Jaeger, S. R., and Traill, W. B. (2004). Objective and subjective knowledge: Impacts on consumer demand for genetically modified foods in the United States and the European Union. AgBioForum 7 (3), 113–123. doi:10.22004/ag.econ.20125
Ikram, M., Sroufe, R., Awan, U., and Abid, N. (2022). Enabling progress in developing economies: A novel hybrid decision-making model for green technology planning. Sustainability 14 (1), 258. doi:10.3390/su14010258
Jansson, J., Nordlund, A., and Westin, K. (2017). Examining drivers of sustainable consumption: The influence of norms and opinion leadership on electric vehicle adoption in Sweden. J. Clean. Prod. 154, 176–187. doi:10.1016/j.jclepro.2017.03.186
Jorgensen, B., Graymore, M., and O'Toole, K. (2009). Household water use behavior: An integrated model. J. Environ. Manage. 91 (1), 227–236. doi:10.1016/j.jenvman.2009.08.009
Joshi, Y., and Rahman, Z. (2017). Investigating the determinants of consumers’ sustainable purchase behaviour. Sustain. Prod. Consumpt. 10, 110–120. doi:10.1016/j.spc.2017.02.002
Kiatkawsin, K., and Han, H. (2017). Young travelers’ intention to behave pro-environmentally: Merging the value-belief-norm theory and the expectancy theory. Tour. Manag. 59, 76–88. doi:10.1016/j.tourman.2016.06.018
Kim, M. S., Kim, J., and Thapa, B. (2018). Influence of environmental knowledge on affect, nature affiliation and pro-environmental behaviors among tourists. Sustain. Switz. 10 (9), 3109. doi:10.3390/su10093109
Lee, J. J., Gemba, K., and Kodama, F. (2006). Analyzing the innovation process for environmental performance improvement. Technol. Forecast. Soc. Change 73 (3), 290–301. doi:10.1016/j.techfore.2004.03.008
Lee, M., Tansel, B., and Balbin, M. (2011). Influence of residential water use efficiency measures on household water demand: A four year longitudinal study. Resour. Conservation Recycl. 56 (1), 1–6. doi:10.1016/j.resconrec.2011.08.006
Levine, D. S., and Strube, M. J. (2012). Environmental attitudes, knowledge, intentions and behaviors among college students. J. Soc. Psychol. 152 (3), 308–326. doi:10.1080/00224545.2011.604363
Liu, Y., Sheng, H., Mundorf, N., Redding, C., and Ye, Y. (2017). Integrating norm activation model and theory of planned behavior to understand sustainable transport behavior: Evidence from China. Int. J. Environ. Res. Public Health 14 (12), 1593. doi:10.3390/ijerph14121593
Lowe, B., Lynch, D., and Lowe, J. (2015). Reducing household water consumption: A social marketing approach. J. Mark. Manag. 31 (3–4), 378–408. doi:10.1080/0267257X.2014.971044
Madias, K., and Szymkowiak, A. (2022). Residential sustainable water usage and water management: Systematic review and future research. Water 14 (7), 1027. doi:10.3390/w14071027
Martono, S., Nurkhin, A., Luthfiyah, F., Fachrurrozie, F., Rofiq, A., and Sumiadji, S. (2019). The relationship between knowledge, trust, intention to pay zakah, and zakah-paying behavior. Int. J. Financial Res. 10 (2), 75–81. doi:10.5430/ijfr.v10n2p75
Montginoul, M., and Vestier, A. (2018). Smart metering: A water-saving solution? Consider communication strategies and user perceptions first. Evidence from a French case study. Environ. Model. Softw. 104, 188–198. doi:10.1016/j.envsoft.2018.02.006
Mostafa, M. M. (2006). Antecedents of Egyptian Consumers’ Green Purchase Intentions. J. Int. Consumer Mark. 19 (2), 97–126. July 2013. doi:10.1300/J046v19n02_06
Onel, N., and Mukherjee, A. (2016). Consumer knowledge in pro-environmental behavior. World J. Sci. Technol. Sustain. Dev. 13, 328–352.
Pakula, C., and Stamminger, R. (2010). Electricity and water consumption for laundry washing by washing machine worldwide. Energy Efficiency 3 (4), 365–382. doi:10.1007/s12053-009-9072-8
Pavalache-Ilie, M., and Unianu, E. M. (2012). Locus of control and the pro-environmental attitudes. Procedia—Social and Behavioral Sciences . 33, 198–202. doi:10.1016/j.sbspro.2012.01.111
Rondinel-Oviedo, D. R., and Sarmiento-Pastor, J. M. (2020). Water: Consumption, usage patterns, and residential infrastructure. A comparative analysis of three regions in the Lima metropolitan area. Water Int. 45 (7–8), 824–846. doi:10.1080/02508060.2020.1830360
Russell, S. V., and Knoeri, C. (2020). Exploring the psychosocial and behavioural determinants of household water conservation and intention. Int. J. Water Resour. Dev. 36 (6), 940–955. doi:10.1080/07900627.2019.1638230
Schumacker, R. E., and Lomax, R. G. (1996). A beginner's guide to structural equation modeling . Hillsdale, NJ, US: Lawrence Erlbaum Associates .
Schwartz, S. H. (1977). Normative influences on altruism. Adv. Exp. Soc. Psychol. 10, 221–279. doi:10.1016/S0065-2601(08)60358-5
Shan, Y., Yang, L., Perren, K., and Zhang, Y. (2015). Household water consumption: Insight from a survey in Greece and Poland. Procedia Eng. 119 (1), 1409–1418. doi:10.1016/j.proeng.2015.08.1001
Shin, Y. H., Im, J., Jung, S. E., and Severt, K. (2018). The theory of planned behavior and the norm activation model approach to consumer behavior regarding organic menus. Int. J. Hosp. Manag. 69, 21–29. doi:10.1016/j.ijhm.2017.10.011
Sønderlund, A. L., Smith, J., Hutton, C., and Kapelan, Z. (2014). Using smart meters for household water consumption feedback: Knowns and unknowns. Procedia Eng. 89, 990–997. doi:10.1016/j.proeng.2014.11.216
Stern, P. C. (2000). New environmental theories: Toward a coherent theory of environmentally significant behavior. J. Soc. Issues 56 (3), 407–424. doi:10.1111/0022-4537.00175
Stern, P. C., Dietz, T., Abel, T., Guagnano, G. A., and kalof, L. (1999). A value-belief-norm theory of support for social movements: The case of environmentalism. Hum. Ecol. Rev. 6 (2), 81–97.
Sulaeman, M., Marhamah, M., Muchtar, A. A., and Ariestiningsih, E. S. (2018). “The role of ascription of responsibility on pro environmental behavior in jakarta communities,” in Proceedings of the 1st International Conference on Recent Innovations , Jakarta, Indonesia , 2251–2258.
Suryanda, A., Sigit, D. V., and Safitri, H. (2021). Relationship between environmental pollution knowledge and green purchase intention of students. AIP Conf. Proc. 2331, 661. doi:10.1063/5.0041661
Vining, J., and Ebreo, A. (2002). Emerging theoretical and methodological perspectives on conservation behavior. Urbana 51.
William, Y., Hwang, K., McDonald, S., and Oates, C. J. (2009). Sustainable consumption: Green consumer behaviour when purchasing products. Sustain. Dev. 18, 20–31. doi:10.1002/sd.394
Willis, R. M., Stewart, R. A., Panuwatwanich, K., Williams, P. R., and Hollingsworth, A. L. (2011). Quantifying the influence of environmental and water conservation attitudes on household end use water consumption. J. Environ. Manag. 92 (8), 1996–2009. doi:10.1016/j.jenvman.2011.03.023
APPENDIX TABLE A1 Survey questions.
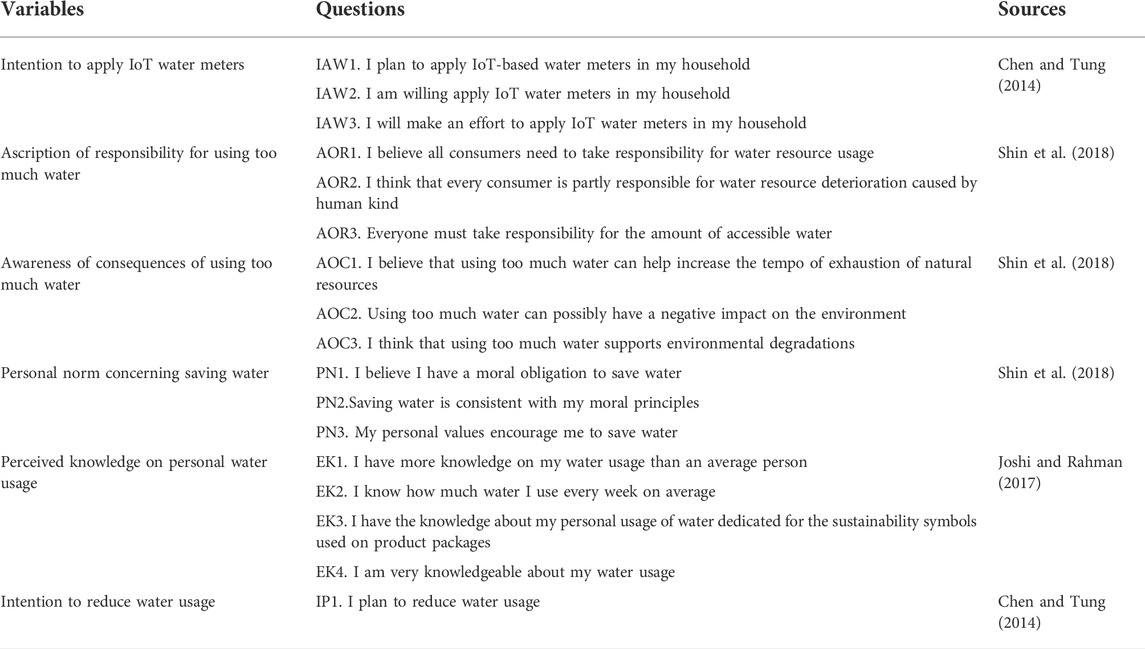
Keywords: IoT, smart meter, water consumption, knowledge, sustainability
Citation: Madias K, Borusiak B and Szymkowiak A (2022) The role of knowledge about water consumption in the context of intentions to use IoT water metrics. Front. Environ. Sci. 10:934965. doi: 10.3389/fenvs.2022.934965
Received: 03 May 2022; Accepted: 26 July 2022; Published: 02 September 2022.
Reviewed by:
Copyright © 2022 Madias, Borusiak and Szymkowiak. This is an open-access article distributed under the terms of the Creative Commons Attribution License (CC BY). The use, distribution or reproduction in other forums is permitted, provided the original author(s) and the copyright owner(s) are credited and that the original publication in this journal is cited, in accordance with accepted academic practice. No use, distribution or reproduction is permitted which does not comply with these terms.
*Correspondence: Konstantinos Madias, [email protected]

An official website of the United States government
The .gov means it’s official. Federal government websites often end in .gov or .mil. Before sharing sensitive information, make sure you’re on a federal government site.
The site is secure. The https:// ensures that you are connecting to the official website and that any information you provide is encrypted and transmitted securely.
- Publications
- Account settings
Preview improvements coming to the PMC website in October 2024. Learn More or Try it out now .
- Advanced Search
- Journal List
- HHS Author Manuscripts

Water, Hydration and Health
Barry m. popkin.
Department of Nutrition, University of North Carolina, Chapel Hill, NC
Kristen E. D’Anci
Department of Psychology, Tufts University, Medford, MA
Irwin H. Rosenberg
Nutrition and Neurocognition Laboratory, Jean Mayer USDA Human Nutrition Research Center on Aging at Tufts University, Boston, MA
This review attempts to provide some sense of our current knowledge of water including overall patterns of intake and some factors linked with intake, the complex mechanisms behind water homeostasis, the effects of variation in water intake on health and energy intake, weight, and human performance and functioning. Water represents a critical nutrient whose absence will be lethal within days. Water’s importance for prevention of nutrition-related noncommunicable diseases has emerged more recently because of the shift toward large proportions of fluids coming from caloric beverages. Nevertheless, there are major gaps in knowledge related to measurement of total fluid intake, hydration status at the population level, and few longer-term systematic interventions and no published random-controlled longer-term trials. We suggest some ways to examine water requirements as a means to encouraging more dialogue on this important topic.
I. INTRODUCTION
Water is essential for life. From the time that primeval species ventured from the oceans to live on land, a major key to survival has been prevention of dehydration. The critical adaptations cross an array of species, including man. Without water, humans can survive only for days. Water comprises from 75% body weight in infants to 55% in elderly and is essential for cellular homeostasis and life. 1 Nevertheless there are many unanswered questions about this most essential component of our body and our diet. This review attempts to provide some sense of our current knowledge of water including overall patterns of intake and some factors linked with intake, the complex mechanisms behind water homeostasis, the effects of variation in water intake on health and energy intake, weight, and human performance and functioning.
Recent statements on water requirements have been based on retrospective recall of water intake from food and beverages among healthy non-institutionalized individuals. We provide examples of water intake assessment in populations to clarify the need for experimental studies. Beyond these circumstances of dehydration, we do not truly understand how hydration affects health and well-being, even the impact of water intakes on chronic diseases. Recently, Jéquier and Constant addressed this question based on the human physiology. 2 We need to know more about the extent that water intake might be important for disease prevention and health promotion.
As we note later, few countries have developed water requirements and those that do base them on weak population-level measures of water intake and urine osmolality. 3 , 4 The European Food Safety Authority (EFSA) has been recently asked to revise existing recommended intakes of essential substances with a physiological effect including water since this nutrient is essential for life and health. 5
The US Dietary Recommendations for water are based on median water intakes with no use of measurements of dehydration status of the population to assist. One-time collection of blood samples for the analysis of serum osmolality has been used by NHANES. At the population level we have no accepted method of assessing hydration status and one measure some scholars use, hypertonicity, is not even linked with hydration in the same direction for all age groups. 6 Urine indices are used often but reflect recent volume of fluid consumed rather than a state of hydration. 7 Many scholars use urine osmolality to measure recent hydration status. 8 – 12 Deuterium dilution techniques (isotopic dilution with D2O or deuterium oxide) allows measurement of total body water but not water balance status. 13 Currently we feel there are no adequate biomarkers to measure hydration status at the population level.
When we speak of water we are essentially focusing first and foremost on all types of water, be they soft or hard, spring or well, carbonated or distilled water. Furthermore we get water not only directly as a beverage but from food and to a very small extent also from oxidation of macronutrients (metabolic water). The proportion of water that comes from beverages and food varies with the proportion of fruits and vegetables in the diet. We present the ranges of water in various foods ( Table 1 ). In the United States it is estimated that about 22% of water comes from our food intake while it would be much higher in European countries, particularly a country like Greece with its higher intake of fruits and vegetables or South Korea. 3 , 14 , 15 The only in-depth study of water use and water intrinsic to food in the US found a 20.7% contribution from food water; 16 , 17 however as we show later, this research was dependent on poor overall assessment of water intake.
The Water Content Range for Selected Foods
Source: The USDA National Nutrient Database for Standard Reference, Release 21 provided in Altman. 127
This review considers water requirements in the context of recent efforts to assess water intake in US populations. Relationship of water and calorie intake is explored both for insights into the possible displacement of calories from sweetened beverages by water and also to examine the possibility that water requirements would be better expressed in relation to calorie/energy requirements with the dependence of the latter on age, size, gender, and physical activity level. We review current understanding of the exquisitely complex and sensitive system which protects land animals against dehydration and comment on the complications of acute and chronic dehydration in man against which a better expression of water requirements might complement the physiological control of thirst. Indeed, the fine intrinsic regulation of hydration and water intake in individuals mitigates against prevalent underhydration in populations and effects on function and disease.
Regulation of fluid intake
To prevent dehydration reptiles, birds, vertebrates, and all land animals have evolved an exquisitely sensitive network of physiological controls to maintain body water and fluid intake by thirst. Humans may drink for various reasons, particularly for hedonic ones but most of drinking is due to water deficiency which triggers the so called regulatory or physiological thirst. The mechanism of thirst is quite well understood today and the reason non-regulatory drinking is often encountered is related to the large capacity of kidneys to rapidly eliminate excesses of water or reduce urine secretion to temporarily economize on water. 1 But this excretory process can only postpone the necessity for drinking or for stopping drinking an excess of water. Non regulatory drinking is often confusing, particularly in wealthy societies facing highly palatable drinks or fluids that contain other substance that the drinker seeks. The most common of them are sweeteners or alcohol to which water is served as a vehicle. Drinking these beverages isn’t due to excessive thirst or hyperdipsia as it can be shown by offering pure water instead and finding out that the same drinker is in fact hypodipsic (Characterized by abnormally diminished thirst). 1
Fluid balance of the two compartments
Maintaining a constant water and mineral balance requires the coordination of sensitive detectors at different sites in the body linked by neural pathways with integrative centers in the brain that process this information. These centers are also sensitive to humoral factors (neurohormones) produced for the adjustment of diuresis, natriuresis and blood pressure (angiotensin mineralocorticoids, vasopressin, atrial natriuretic factor). Instructions from the integrative centers to the “executive organs” (kidney, sweat glands and salivary glands) and to the part of the brain responsible for corrective actions such as drinking are conveyed by certain nerves in addition to the above mentioned substances. 1
Most of the components of fluid balance are controlled by homeostatic mechanisms responding to the state of body water. These mechanisms are sensitive and precise, and are activated with deficits or excesses of water amounting to only a few hundred milliliters. A water deficit produces an increase in the ionic concentration of the extracellular compartment, which takes water from the intracellular compartment causing cells to shrink. This shrinkage is detected by two types of brain sensors, one controlling drinking and the other controlling the excretion of urine by sending a message to the kidneys mainly via the antidiuretic hormone vasopressin to produce a smaller volume of more concentrated urine. 18 When the body contains an excess of water, the reverse processes occur: the lower ionic concentration of body fluids allows more water to reach the intracellular compartment. The cells imbibe, drinking is inhibited and the kidneys excrete more water.
The kidneys thus play a key role in regulating fluid balance. As discussed later, the kidneys function more efficiently in the presence of an abundant water supply. If the kidneys economize on water, producing a more concentrated urine, these is a greater cost in energy and more wear on their tissues. This is especially likely to occur when the kidneys are under stress, for example when the diet contains excessive amounts of salt or toxic substances that need to be eliminated. Consequently, drinking enough water helps protect this vital organ.
Regulatory drinking
Most drinking obeys signals of water deficit. Apart from urinary excretion, the other main fluid regulatory process is drinking, mediated through the sensation of thirst. There are two distinct mechanisms of physiological thirst: the intracellular and the extracellular mechanisms. When water alone is lost, ionic concentration increases. As a result, the intracellular space yields some of its water to the extracellular compartment. One again, the resulting shrinkage of cells is detected by brain receptors that send hormonal messages to induce drinking. This association with receptors that govern extracellular volume is therefore accompanied by an enhancement of salt appetite. Thus, people who have been sweating copiously prefer drinks that are relatively rich in Na+ salts rather than pure water. As previously mentioned, it is always important to supplement drinks with additional salt when excessive sweating is experienced.
The brain’s decision to start or stop drinking and to choose the appropriate drink is made before the ingested fluid can reach the intra- and extracellular compartments. The taste buds in the mouth send messages to the brain about the nature, and especially the salt of the ingested fluid, and neuronal responses are triggered as if the incoming water had already reached the bloodstream. These are the so-called anticipatory reflexes: they cannot be entirely “cephalic reflexes” because they arise from the gut as well as the mouth. 1
The anterior hypothalamus and pre-optic area are equipped with osmo-receptors related to drinking. Neurons in these regions show enhanced firing when the inner milieu gets hyperosmotic. Their firing decreases when water is loaded in the carotid artery that irrigates the neurons. It is remarkable that the same decrease in firing in the same neurons takes place when the water load is applied on the tongue instead of being injected in the carotid artery. This anticipatory drop in firing is due to a mediation neural pathways departing from the mouth and by converging on to the neurons which simultaneously sense of the inner milieu (blood).
Non-regulatory drinking
Although everyone experiences thirst from time to time, it plays little day-to-day role in the control of water intake in healthy people living in temperate climates. We generally consume fluids not to quench our thirst, but as components of everyday foods (e.g. soup, milk), as beverages used as mild stimulants (tea, coffee) and for pure pleasure. As common example is alcohol consumption which can increase individual pleasure and stimulate social interaction. Drinks are also consumed for their energy content, as in soft drinks and milk, and are used in warm weather for cooling and in cold weather for warming. Such drinking seems also to be mediated through the taste buds, which communicate with the brain in a kind of “reward system” the mechanisms of which are just beginning to be understood. This bias in the way human beings rehydrate themselves may be advantageous because it allows water losses to be replaced before thirst-producing dehydration takes place. Unfortunately, this bias also carries some disadvantages. Drinking fluids other than water can contribute to an intake of caloric nutrients in excess of requirements, or in alcohol consumption that in some people may insidiously bring about dependence. For example, total fluid intake increased from 79 fluid ounces in 1989 to 100 fluid ounces in 2002 among US adults, all from caloric beverages. 19
Effects of aging on fluid intake regulation
The thirst and fluid ingestion responses of older persons to a number of stimuli have been compared to those seen in younger persons. 20 Following water deprivation older persons are less thirsty and drink less fluid compared to younger persons. 21 , 22 The decrease in fluid consumption is predominantly due to a decrease in thirst as the relationship between thirst and fluid intake is the same in young and old persons. Older persons drink insufficient water following fluid deprivation to replenish their body water deficit. 23 When dehydrated older persons are offered a highly palatable selection of drinks, this also failed to result in an increased fluid intake. 23 The effects of increased thirst in response to an osmotic load have yielded variable responses with one group reporting reduced osmotic thirst in older individuals 24 and one failing to find a difference. In a third study, young individuals ingested almost twice as much fluid as old persons, despite the older subjects having a much higher serum osmolality. 25
Overall these studies support small changes in the regulation of thirst and fluid intake with aging. Defects in both osmoreceptors and baroreceptors appear to exist as well as changes in the central regulatory mechanisms mediated by opioid receptors. 26 Because of their low water reserves, it may be prudent for the elderly to learn to drink regularly when not thirsty and to moderately increase their salt intake when they sweat. Better education on these principles may help prevent sudden hypotension and stroke or abnormal fatigue can lead to a vicious circle and eventually hospitalization.
Thermoregulation
Hydration status is critical to the body’s process of temperature control. Body water loss through sweat is an important cooling mechanism in hot climates and in physical activity. Sweat production is dependent upon environmental temperature and humidity, activity levels, and type of clothing worn. Water losses via skin (both insensible perspiration and sweating) can range from 0.3 L/h in sedentary conditions to 2.0 L/h in high activity in the heat and intake requirements range from 2.5 to just over 3 L/d in adults under normal conditions, and can reach 6 L/d with high extremes of heat and activity. 27 , 28 Evaporation of sweat from the body results in cooling of the skin. However, if sweat loss is not compensated for with fluid intake, especially during vigorous physical activity, a hypohydrated state can occur with concomitant increases in core body temperature. Hypohydration from sweating results in a loss in electrolytes, as well as a reduction in plasma volume, and can lead to increased plasma osmolality. During this state of reduced plasma volume and increased plasma osmolality, sweat output becomes insufficient to offset increases in core temperature. When fluids are given to maintain euhydration, sweating remains an effective compensation for increased core temperatures. With repeated exposure to hot environments, the body adapts to heat stress, and cardiac output and stroke volume return to normal, sodium loss is conserved, and the risk for heat-stress related illness is reduced. 29 Increasing water intake during this process of heat acclimatization will not shorten the time needed to adapt to the heat, but mild dehydration during this time may be of concern and is associated with elevations in cortisol, increased sweating, and electrolyte imbalances. 29
Children and the elderly have differing responses to ambient temperature and different thermoregulatory concerns than healthy adults. Children in warm climates may be more susceptible to heat illness than adults due to greater surface area to body mass ratio, lower rate of sweating, and slower rate of acclimatization to the heat. 30 , 31 Children may respond to hypohydration during activity with a higher relative increase in core temperature than adults do, 32 and sweat less, thus losing some of the benefits of evaporative cooling. However, it has been argued that children can dissipate a greater proportion of body heat via dry heat loss, and the concomitant lack of sweating provides a beneficial means of conserving water under heat stress. 30 Elders, in response to cold stress, show impairments in thermoregulatory vasoconstriction and body water is shunted from plasma into the interstitial and intracellular compartments. 33 , 34 With respect to heat stress, water lost through sweating decreases water content of plasma, and the elderly are less able to compensate for increased blood viscosity. 33 Not only do they have a physiological hypodipsia, but this can be exaggerated by central nervous system disease 35 and by dementia 36 . In addition, illness and limitations in activities of daily living can further limit fluid intake. Coupled with reduced fluid intake, with advancing age there is a decrease in total body water. Older individuals have impaired renal fluid conservation mechanisms and, as noted above, have impaired responses to heat and cold stress 33 , 34 . All of these factors contribute to an increased risk of hypohydration and dehydration in the elderly.
II. PHYSIOLOGICAL EFFECTS OF DEHYDRATION
In this section, the role of water in health is generally characterized in terms of deviations from an ideal hydrated state, generally in comparison to dehydration. The concept of dehydration encompasses both the process of losing body water and also the state of dehydration. Much of the research on water and physical or mental functioning compares a euhydrated state, usually achieved by provision of water sufficient to overcome water loss, to a dehydrated state, which is achieved via withholding of fluids over time and during periods of heat stress or high activity. In general, provision of water is beneficial in those with a water deficit, but little research supports the notion that additional water in adequately hydrated individuals confers any benefit.
Physical performance
The role of water and hydration in physical activity, particularly in athletes and in the military, has been of considerable interest and is well-described in the scientific literature. 37 – 39 During challenging athletic events, it is not uncommon for athletes to lose 6–10% of body weight in sweat loss, thus leading to dehydration if fluids have not been replenished. However, decrements in physical performance in athletes have been observed under much lower levels of dehydration, as little as 2%. 38 Under relatively mild levels of dehydration, individuals engaging in rigorous physical activity will experience decrements in performance related to reduced endurance, increased fatigue, altered thermoregulatory capability, reduced motivation, and increased perceived effort. 40 , 41 Rehydration can reverse these deficits, and also reduce oxidative stress induced by exercise and dehydration. 42 Hypohydration appears to have a more significant impact on high-intensity and endurance activity such as tennis 43 and long-distance running 44 than on anaerobic activities 45 such as weight lifting or on shorter-duration activities, such as rowing. 46
During exercise, individuals may not hydrate adequately when allowed to drink according to thirst. 32 After periods of physical exertion, voluntary fluid intake may be inadequate to offset fluid deficits. 1 Thus, mild to moderate dehydration can therefore persist for some hours after the conclusion of physical activity. Research in athletes suggests that, principally at the beginning of the season, they are at particular risk for dehydration due to lack of acclimatization to weather conditions or suddenly increased activity levels. 47 , 48 A number of studies show that performance in temperate and hot climates is affected to a greater degree than performance in cold temperatures. 41 , 49 , 50 Exercise in hot conditions with inadequate fluid replacement is associated with hyperthermia, reduced stroke volume and cardiac output, decreases in blood pressure, and reduced blood flow to muscle. 51
During exercise, children may be at greater risk for voluntary dehydration. Children may not recognize the need to replace lost fluids, and both children as well as coaches need specific guidelines for fluid intake. 52 Additionally, children may require longer acclimation to increases in environmental temperature than do adults. 30 , 31 Recommendations are for child athletes or children in hot climates to begin athletic activities in a well-hydrated state and to drink fluids over and above the thirst threshold.
Cognitive performance
Water, or its lack (dehydration), can influence cognition. Mild levels of dehydration can produce disruptions in mood and cognitive functioning. This may be of special concern in the very young, very old, those in hot climates, and those engaging in vigorous exercise. Mild dehydration produces alterations in a number of important aspects of cognitive function such as concentration, alertness and short-term memory in children (10–12 y), 32 young adults (18–25y) 53 – 56 and in the oldest adults, 50–82y. 57 As with physical functioning, mild to moderate levels of dehydration can impair performance on tasks such as short-term memory, perceptual discrimination, arithmetic ability, visuomotor tracking, and psychomotor skills. 53 – 56 However, mild dehydration does not appear to alter cognitive functioning in a consistent manner. 53 , 54 , 56 , 58 In some cases, cognitive performance was not significantly affected in ranges from 2–2.6% dehydration. 56 , 58 Comparing across studies, performance on similar cognitive tests was divergent under dehydration conditions. 54 , 56 In studies conducted by Cian and colleagues, 53 , 54 participants were dehydrated to approximately 2.8% either through heat exposure or treadmill exercise. In both studies, performance was impaired on tasks examining visual perception, short-term memory, and psychomotor ability. In a series of studies using exercise in conjunction with water restriction as a means of producing dehydration, D’Anci and colleagues 56 observed only mild decrements in cognitive performance in healthy young men and women athletes. In these experiments, the only consistent effect of mild dehydration was significant elevations of subjective mood score, including fatigue, confusion, anger, and vigor. Finally, in a study using water deprivation alone over a 24-h period, no significant decreases in cognitive performance were seen with 2.6% dehydration 58 . It is possible therefore, that heat-stress may play a critical role in the effects of dehydration on cognitive performance.
Reintroduction of fluids under conditions of mild dehydration can reasonably be expected to reverse dehydration-induced cognitive deficits. Few studies have examined how fluid reintroduction may alleviate dehydration’s negative effects on cognitive performance and mood. One study 59 examined how water ingestion affected arousal and cognitive performance in young people following a period of 12-h water restriction. While cognitive performance was not affected by either water restriction or water consumption, water ingestion affected self-reported arousal. Participants reported increased alertness as a function of water intake. Rogers and coworkers 60 observed a similar increase in alertness following water ingestion in both high- and low-thirst participants. Water ingestion, however, had opposite effects on cognitive performance as a function of thirst. High-thirst participants’ performance on a cognitively demanding task improved following water ingestion, but low-thirst participants’ performance declined. In summary, hydration status consistently affected self-reported alertness, but effects on cognition were less consistent.
Several recent studies have examined the utility of providing water to school children on attentiveness and cognitive functioning in children. 61 – 63 In these experiments, children were not fluid restricted prior to cognitive testing, but were allowed to drink as usual. Children were then provided with a drink or no drink 20–45 minutes before the cognitive test sessions. In the absence of fluid restriction and without physiological measures of hydration status, the children in these studies should not be classified as dehydrated. Subjective measures of thirst were reduced in children given water, 62 and voluntary water intake in children varied from 57 ml to 250 ml. In these studies, as in the studies in adults, the findings were divergent and relatively modest. In the research led by Edmonds and colleagues, 61 , 62 children in the groups given water showed improvements in visual attention. However, effects on visual memory were less consistent, with one study showing no effects of drinking water on a spot-the-difference task in 6–7 year old children 61 and the other showing a significant improvement in a similar task in 7–9 year old children 62 In the research described by Benton and Burgess, 63 memory performance was improved by provision of water but sustained attention was not altered with provision of water in the same children.
Taken together these studies indicate that low to moderate dehydration may alter cognitive performance. Rather than indicating that the effects of hydration or water ingestion on cognition are contradictory, many of the studies differ significantly in methodology and in measurement of cognitive behaviors. These variances in methodology underscore the importance of consistency when examining relatively subtle chances in overall cognitive performance. However, in those studies in which dehydration were induced, most combined heat and exercise, thus it is difficult to disentangle the effects of dehydration on cognitive performance in temperate conditions, from the effects of heat and exercise. Additionally, relatively little is known about the mechanism of mild dehydration’s effects on mental performance. It has been proposed that mild dehydration acts as a physiological stressor which competes with and draws attention from cognitive processes 64 . However, research on this hypothesis is limited and merits further exploration.
Dehydration and delirium
Dehydration is a risk factor for delirium and delirium presenting as dementia in the elderly and in the very ill. 65 – 67 Recent work shows that dehydration is one of several predisposing factors in observed confusion in long-term care residents, 67 although in this study daily water intake was used as a proxy measure for dehydration rather than other, more direct clinical assessments such as urine or plasma osmolality. Older people have been reported as having reduced thirst and hypodypsia relative to younger people. In addition, fluid intake and maintenance of water balance can be complicated by factors such as disease, dementia, incontinence, renal insufficiency, restricted mobility, and drug side effects. In response to primary dehydration, older people have less thirst sensation and reduced fluid intakes in comparison to younger people. However, in response to heat stress, while older people still display a reduced thirst threshold, they do ingest comparable amounts of fluid as younger people. 20
Gastrointestinal function
Fluids in the diet are generally absorbed in the proximal small intestine, and absorption rate is determined by the rate of gastric emptying to the small intestine. Therefore, the total volume of fluid consumed will eventually be reflected in water balance, but the rate at which rehydration occurs is dependent upon factors which affect the rate of delivery of fluids to the intestinal mucosa. Gastric emptying rate is generally accelerated by the total volume consumed and slowed by higher energy density and osmolality. 68 In addition to water consumed in food (1 L/d) and beverages (~2–3 L/d), digestive secretions account for a far greater portion of water that passes through and is absorbed by the gastrointestinal tract (~8 L/d). 69 The majority of this water is absorbed by the small intestine, with a capacity of up to 15 L/d with the colon absorbing some 5 L/d. 69
Constipation, characterized by slow gastrointestinal transit, small, hard stools, and difficulty in passing stool, has a number of causes including medication use, inadequate fiber intake, poor diet, and illness. 70 Inadequate fluid consumption is touted as a common culprit in constipation, and increasing fluid intake is a frequently recommended treatment. Evidence suggests, however, that increasing fluids is only of usefulness in individuals in a hypohydrated state, and is of little utility in euhydrated individuals. 70 In young children with chronic constipation, increasing daily water intake by 50% did not affect constipation scores. 71 For Japanese women with low fiber intake, concomitant low water intake in the diet is associated with increased prevalence of constipation. 72 In older individuals, low fluid intake is a predictor for increased levels of acute constipation 73 , 74 with those consuming the least amount of fluid having over twice the frequency of constipation episodes than those consuming the most fluid. In one trial, researchers compared the utility of carbonated mineral water in reducing functional dyspepsia and constipation scores to tap water in individuals with functional dyspepsia. 75 When comparing carbonated mineral water to tap water, participants reported improvements in subjective gastric symptoms, but there were no significant improvements in gastric or intestinal function. The authors indicate that it is not possible to determine to what degree the mineral content of the two waters contributed to perceived symptom relief, as the mineral water contained greater levels of magnesium and calcium than the tap water. The available evidence suggests that increased fluid intake should only be indicated in individuals in a hypohydrated state. 71 , 69
Significant water loss can occur through the gastrointestinal tract, and this can be of great concern in the very young. In developing countries, diarrheal diseases are a leading cause of death in children resulting in approximately 1.5–2.5 million deaths per year. 76 Diarrheal illness results not only in a reduction in body water, but also in potentially lethal electrolyte imbalances. Mortality in such cases can many times be prevented with appropriate oral rehydration therapy, by which simple dilute solutions of salt and sugar in water can replace fluid lost by diarrhea. Many consider application of oral rehydration therapy to be one of the signal public health developments of the last century. 77
Kidney function
As noted above, the kidney is crucial in regulating water balance and blood pressure as well as removing waste from the body. Water metabolism by the kidney can be classified into regulated and obligate. Water regulation is hormonally mediated, with the goal of maintaining a tight range of plasma osmolality between 275 to 290 mOsm/kg. Increases in plasma osmolality, and activation of osmoreceptors (intracellular) and baroreceptors (extracellular) stimulate hypothalamic release of arginine vasopressin (AVP). AVP acts at the kidney to decrease urine volume and promote retention of water, and the urine becomes hypertonic. With decreased plasma osmolality, vasopressin release is inhibited, and the kidney increases hypotonic urinary output.
In addition to regulating fluid balance, the kidneys require water for the filtration of waste from the blood stream and excretion via urine. Water excretion via the kidney removes solutes from the blood, and a minimum obligate urine volume is required to remove the solute load with a maximum output volume of 1 L/h. 78 This obligate volume is not fixed, but is dependent upon the amount of metabolic solutes to be excreted and levels of AVP. Depending on the need for water conservation, basal urine osmolality ranges from 40 mOsm/kg up to a maximum of 1400 mOsm/kg. 78 The ability to both concentrate and dilute urine decreases with age, with a lower value of 92 mOsm/kg and an upper range falling between 500–700 mOsm/kg for individuals over 70. 79 – 81 Under typical conditions, in an average adult, urine volume of 1.5 to 2.0 L/d would be sufficient to clear a solute load of 900 to 1200 mOsm/d. During water conservation and the presence of AVP, this obligate volume can decrease to 0.75–1.0 L/d and during maximal diuresis can require up to 20 L/d to remove the same solute load. 78 , 80 , 81 In cases of water loading, if the volume of water ingested cannot be compensated for with urine output, having overloaded the kidney’s maximal output rate, an individual can enter a hyponatremic state as described above.
Heart function and hemodynamic response
Blood volume, blood pressure, and heart rate are closely linked. Blood volume is normally tightly regulated by matching water intake and water output, as described in the section on kidney function. In healthy individuals, slight changes in heart rate and vasoconstriction act to balance the effect of normal fluctuations in blood volume on blood pressure. 82 Decreases in blood volume can occur, through blood loss (or blood donation), or loss of body water through sweat, as seen with exercise. Blood volume is distributed differently relative to the position of the heart whether supine or upright, and moving from one position to the other can lead to increased heart rate, a fall in blood pressure and, in some cases, lead to syncope. This postural hypotension (or orthostatic hypotension) can be mediated by drinking 300–500 ml of water. 83 , 84 Water intake acutely reduces heart rate and increases blood pressure in both normotensive and hypertensive individuals. 85 These effects of water intake on the pressor effect and heart rate occur within 15–20 minutes of drinking water and can last for up to 60 minutes. Water ingestion is also beneficial in preventing vasovagal reaction with syncope in blood donors at high risk for post-donation syncope. 86 The effect of water drinking in these situations is thought to be due to effects on the sympathetic nervous system rather than to changes in blood volume. 83 , 84 Interestingly, in rare cases, individuals may experience bradycardia and syncope after swallowing cold liquids. 87 – 89 While swallow syncope can be seen with other substances than water, swallow syncope further supports the notion that the result of water ingestion in the pressor effect has both a neural component as well as a cardiac component.
Water deprivation and dehydration can lead to the development of headache. 90 Although this observation is largely unexplored in the medical literature, some observational studies indicate that water deprivation, in addition to impairing concentration and increasing irritability, can serve as a trigger for migraine and also prolong migraine. 91 , 92 In those with water deprivation-induced headache, ingestion of water provided relief from headache in most individuals within 30 min to 3 h. 92 It is proposed that water deprivation-induced headache is the result of intracranial dehydration and total plasma volume. Although provision of water may be useful in relieving dehydration related headache, the utility of increasing water intake for the prevention of headache is less well documented.
The folk wisdom that drinking water can stave off headaches has been relatively unchallenged, and has more traction in the popular press than in the medical literature. Recently, one study examined increased water intake and headache symptoms in headache patients. 93 In this randomized trial, patients with a history of different types of headache, including migraine and tension headache, were either assigned to a placebo condition (a non-drug tablet) or the increased water condition. In the water condition, participants were instructed to consume an additional volume of 1.5 L water/day on top of what they already consumed in foods and fluids. Water intake did not affect number of headache episodes, but was modestly associated with reduction in headache intensity and reduced duration of headache. The data from this study suggest that water is limited as prophylaxis in headache sufferers, and the ability of water to reduce or prevent headache in a broader population remains unknown.
One of the more pervasive myths regarding water intake is the improvement of the skin or complexion. By improvement, it is generally understood that individuals are seeking to have a more “moisturized” look to the surface skin, or to minimize acne or other skin conditions. Numerous lay sources such as beauty and health magazines as well as the Internet suggest that drinking 8–10 glasses of water a day will “flush toxins from the skin” and “give a glowing complexion” despite a general lack of evidence 94 , 95 to support these proposals. The skin, however, is important in maintaining body water levels and preventing water loss into the environment.
The skin contains approximately 30% water, which contributes to plumpness, elasticity, and resiliency. The overlapping cellular structure of the stratum corneum and lipid content of the skin serves as “waterproofing” for the body. 96 Loss of water through sweat is not indiscriminate across the total surface of the skin, but is carried out by eccrine sweat glands, which are evenly distributed over most of the body surface. 97 Skin dryness is usually associated with exposure to dry air, prolonged contact with hot water and scrubbing with soap (both strip oils from the skin), medical conditions and medications. While more serious levels of dehydration can be reflected in reduced skin turgor, 98 , 99 with tenting of the skin as a flag for dehydration, overt skin turgor in individuals with adequate hydration is not altered. Water intake, particularly in individuals with low initial water intake, can improve skin thickness and density as measured by sonogram, 100 and offsets transepidermal water loss, and can improve skin hydration. 101 Adequate skin hydration, however, is not sufficient to prevent wrinkles or other signs of aging, which are related to genetics, and sun and environmental damage. Of more utility to individuals already consuming adequate fluids, the use of topical emollients will improve skin barrier function and improve the look and feel of dry skin. 102 , 103
Hydration and chronic diseases
Many chronic diseases have multifactorial origins. In particular, differences in lifestyle and the impact of environment are known to be involved and constitute risk factors that are still being evaluated. Water is quantitatively the most important nutrient. In the past, scientific interest with regard to water metabolism was mainly directed toward the extremes of severe dehydration and water intoxication. There is evidence, however, that mild dehydration may also account for some morbidities. 4 , 104 There is currently no consensus on a “gold standard” for hydration markers, particularly for mild dehydration. As a consequence, the effects of mild dehydration on the development of several disorders and diseases have not been well documented.
There is strong evidence showing that good hydration reduces the risk of urolithiasis (See Table 2 for evidence categories). Less strong evidence links good hydration with reduced incidence of constipation, exercise asthma, hypertonic dehydration in the infant, and hyperglycemia in diabetic ketoacidosis. Good hydration is associated with a reduction in urinary tract infections, hypertension, fatal coronary heart disease, venous thromboembolism, and cerebral infarct but all these effects need to be confirmed by clinical trials. For other conditions such as bladder or colon cancer, evidence of a preventive effect of maintaining good hydration is not consistent (see Table 3 ).
Categories of evidence in evaluating the quality of reports
Adapted from Manz 104
Hydration Status and Chronic Diseases
III. Water consumption and requirements and relationships to total energy intake
Water consumption, water requirements and energy intake are linked in fairly complex ways. This is partially because physical activity and energy expenditures affect the need for water but also because a large shift in beverage consumption over the past century or more has led to consumption of a significant proportion of our energy intake from caloric beverages. Nonregulatory beverage intake, as noted earlier, has assumed a much greater role for individuals. 19 In this section we first review current patterns of water intake, then refer to a full meta-analysis of the effects of added water on energy intake. This includes adding water to the diet and water replacement for a range of caloric and diet beverages, including sugar-sweetened beverages, juice, milk, and diet beverages. The third component is a discussion of water requirements and suggestions for considering the use of ml water/kcal energy intake as a metric.
A. Patterns and trends of water consumption
Measurement of total fluid water consumption in free-living individuals is fairly new in focus. As a result, the state of the science is poorly developed, data are most likely fairly incomplete, and adequate validation of the measurement techniques used are not available. We first present varying patterns and trends of water intake for the United States over the past three decades and review briefly the work on water intake in Europe.
We have really no information to allow us to assume that consumption of water alone or beverage containing water affects hydration differentially. 3 , 105 Some epidemiological data suggests water might have differential metabolic effects when consumed as water alone rather than water contained in caffeinated or flavored or sweetened beverages but these data are at best suggestive of an issue deserving of exploration. 106 , 107 We do show below from the research of Ershow that beverages not consisting of solely water do contain less than 100% water.
One study in the United States has attempted to examine all the sources of water in our diet. 16 , 17 These data are cited in Table 4 as the Ershow study and were based on National Food Consumption Survey food and fluid intake data from 1977–78. These data are presented in Table 4 for children 2–18 (Panel A) and for adults 19 and older (Panel B). Ershow and colleagues spent a great deal of time working out ways to convert USDA dietary data into water intake, including water absorbed during the cooking process, water in food, and all sources of drinking water. 16 , 17
Beverage Pattern Trends in the United States for Children aged 2–18 and Adults Aged 19 and older, Nationally Representative
Note: The data are age and sex adjusted to 1965; for 1977–78 the 488, 95, and 736 come from the Ershow calculations.
These created a number of categories and used a range of factors measured in other studies to estimate the water categories. The water that is found in food, based on food composition table data, was 393ml for children. The water that was added as a result of cooking (e.g., rice) was 95 ml. That consumed as a beverage directly as water was 624 ml. The water found in other fluids as noted comprised the remainder of the ml with the most water coming from whole fat milk and juices (506 ml). There is a small discrepancy between the Ershow total fluid intake measures for these children and that of the normal USDA figure. That is because USDA does not remove milk fats and solids, fiber and other food constituents found in beverages, particularly, juice and milk.
A key point to be seen in these nationally representative US data is enormous variability in the amount of water consumed between survey waves (see Figure 1 which highlights that large variation in water intake measured in these surveys). Although adult and child water intake moved up and down at the same time, for reasons we cannot explain, the variation is greater among children than adults. This is partly that the questions asked have varied and there has not been detailed probing for water intake as the focus has been on obtaining measures of macro-and micronutrients. Dietary survey methods in the past have focused on obtaining foods and beverages containing nutrient and nonnutritive sweeteners but not on water. Related are the huge differences between the NHANES 1988–1994 and 1999 and later surveys and the USDA surveys. In addition even the NHANES1999–2002 and the 2003–6 surveys differ greatly. This represents a shift in mode of questioning to inclusion of water intake as part of a standard 24-hour recall rather than as standalone questions. Water was not even measured in 1965, and the way the questions have been asked and the limitation on probes for water intake are very clear from a review of the questionnaires plus these data. Essentially in the past people were asked how much water they consumed in a day and now they are asked these questions as part of a 24-hour recall survey. However, unlike other caloric and diet beverages, there are limited probes for water. These must be viewed as crude approximations of total water intake without any strong research to show if they are over- or underestimated. We know from our own research with several studies of water and two on-going random controlled trials that probes that include consideration of all beverages including water as a separate item provide more complete data.

Water Consumption Trends from USDA and NHANES Surveys (ml/day/capita), weighted to be nationally representative
Note: this includes water from fluids only, excluding water in foods. Sources for 1965, 77–78, 89- are USDA. Others are NHANES and 2005–6 is joint USDA and NHANES
Water consumption data for Europe are collected far more selectively than even the crude water intake questions from NHANES. The recent EFSA report provides measures of water consumption from a range of studies in Europe. 4 , 105 , 108 , 109 Essentially what these studies show is that total water intake is lower across Europe than the United States. As with the United States data, none are based on long-term carefully measured or even repeated 24-hour recall measures of water intake from food and beverages. In unpublished work Popkin and Jebb 110 are examining water intake in UK adults in 1986–87 and 2001–2. Their intake increased by 226 ml/d over this time period but still is only 1787 ml/d in the latter period, far below the US figure for 2005–6 of 2793 or earlier figures for comparably aged adults.
There are a few studies in the US and Europe that utilize 24-hour urine and serum osmolality measures to determine total water turnover and this measure of hydration status. These studies suggest that US adults consume over 2100 ml of water per day while those from Europe consume less than a half liter or more. 4 , 111 Data on total urine collection would appear to be another useful measure for examining total water intake. Of course few studies aside from the Donald adolescent cohort in Germany have collected such data on population levels for large samples. 109
B. The effects of water consumption on overall energy intake
There is an extensive literature that focuses on the impact of sugar-sweetened beverages on weight and risk of obesity, diabetes and heart disease; however the perspective of providing more water and its impact on health has not been examined. The water literature does not address portion sizes but rather focuses mainly on water ad libitum or in selected portions compared with other caloric beverages. Elsewhere we have prepared a detailed meta-analysis of the effects of water intake alone (adding additional water), replacing sugar-sweetened beverages, juice, milk and diet beverages. 112
In general, the results of this review suggest that water, when replacing sugar-sweetened beverages, juice and milk is linked with reduced energy intake. This comes mainly from the clinical feeding studies but also from one very good random controlled school intervention and several other epidemiological and intervention studies. Aside from portion sizes, there are issues of timing of the beverage intake and meals (delay time from the beverage to the meal) and types of caloric sweeteners that remain to be considered. However when beverages are consumed in a normal free-living situations where 5–8 eating occasions are the norm, the delay time from the beverage to the meal may matter less. 113 – 115
The literature on children is extremely limited as it relates to water intake. However, the excellent German school intervention with water would suggest the effects of water on overall energy intake of children might be comparable to that of adults. 116 In this German study children were educated on the value of water and provided in school with special filtered drinking fountains and water bottles. The intervention school children increased by 1.1 glasses/day (P<0.001) and reduced their risk of overweight by 31% (OR=0.69, P=0.40).
C. Water requirements: Evaluation of the adequacy of water intake
Classically, water data are examined in terms of milliliters (or some other measure of water volume consumed per capita per day by age group). This measure does not link fluid and caloric intake. Disassociation of fluid and calorie intake difficult for clinicians dealing with an older person who has reduced caloric intake. This ml water measure assumes some mean body size (or surface area) and a mean level of physical activity – both determinants of not only energy expenditure, but also of water balance. Children are dependent on adults for access to water and studies suggest that a larger surface area to volume ratio makes them susceptible to changes in skin temperatures, linked with ambient temperature shifts. 117 One option utilized by some scholars is to explore in ml/kcal of food and beverage intake as was done in the 1989 US RDAs. 4 , 118 This is an option interpretable for clinicians and does incorporate in some sense body size or surface area and activity. It has one disadvantage, namely that water as consumed with caloric beverages, affects both the numerator and denominator; however we do not know a measure that could be independent of this direct effect on body weight and/or total caloric intake.
Despite its critical importance in health and nutrition, as noted earlier, the array of available research that serves as a basis for determining requirements for water or fluid intake, or even rational recommendations for populations, is limited compared to most other nutrients. While this deficit may be partly explained by the highly sensitive set of neurophysiological adaptations and adjustments that occur over a large range of fluid intake to protect body hydration and osmolarity, this deficit remains a challenge for the nutrition and public health community. The latest official effort at recommending water intake for different subpopulations was a part of the Dietary Reference Intake process of the Institute of Medicine of the National Academy of Science’s Report on Dietary Reference Intakes on Water and Electrolytes as reported in 2005. 3 As a graphic acknowledgement of the limited database upon which to express Estimated Average Requirements for water for different population groups, the Committee and the Institute of Medicine were forced to state “While it might appear useful to estimate an average requirement (an EAR) for water, an EAR based on data is not possible”. Given the extreme variability in water needs that are not solely based on differences in metabolism, but also on environmental conditions and activities, there is not a single level of water intake that would assure adequate hydration and optimum health for half of all apparently healthy persons in all environmental conditions. Thus, an Adequate Intake (AI) is established in place of an EAR for water.
The AI for different population groups was set as the median water intake in populations from the National Health and Nutrition Examination Survey, whose intake levels varied greatly based on the survey years (e.g., NHANES 1988–94 vs NHANES 1999–2002) and also were much higher than the USDA surveys (e.g., 1989–91, 1994–98, or 2005–6). If the AI for adults as expressed in Table 5 is taken as a recommended intake, we question the wisdom of the conversion of an AI into recommended water or fluid intake. The first problem is the almost certain inaccuracy of the fluid intake information from the national surveys, even though that problem may also exist for other nutrients. More importantly, from the standpoint of translating an AI into recommended fluid intake for individuals or populations, is the decision in setting the AI to add an additional roughly 20% of water intake, which is derived from some foods in addition to water and beverages. While this may be a legitimate effort to express total water intake as a basis for setting the AI, the recommendations that derive from this IOM report would better be directed at recommendations for water and other fluid intake on the assumption that the water content of foods would be a “passive” addition to total water intake. In this case, the observations of the DRI committee that water intake needs to meet needs imposed by not only metabolism and environmental conditions, but also body size, gender and physical activity. Those are the well studied factors which allow a rather precise measurement and determination of energy intake requirements. It is only logical that those same factors might underlie recommendations to meet water intake needs in the same populations and individuals, and therefore that consideration be given and data gathering be done by experimental and population research, to the possibility that water intake needs would best be expressed relative to the calorie requirements, as is done regularly in the clinical setting.
Water requirements expressed in relation to energy recommendations
AI for total fluids derived from dietary reference intakes for water, potassium, sodium, chloride, and sulfate
Ratios for water intake based on the AI for water in liters/day calculated using EER for each range of physical activity. EER adapted from the Institute of Medicine Dietary Reference Intakes Macronutrients Report, 2002.
It is important to note that only a few countries even include water on the list of nutrients. 119 The European Food Safety Authority is developing a European wide standard. 105 At present only the United States and Germany provide Adequate intake (AI) values but no other country does that. 3 , 120
Another way of considering an approach to the estimation of water requirements beyond the limited usefulness of the AI or estimated mean intake is to express water intake requirements in relation to energy requirements in ml/kcal. An argument for this approach includes the observation that energy requirements are strongly evidence-based in each age and gender group on extensive research which takes into account body size, and activity level which are crucial determinants of energy expenditure which must be met by dietary energy intake. Such measures of expenditure have used highly accurate methods such as doubly-labeled water and thus EERs (Estimated Energy Requirements) have been set based on solid data rather than the compromise inherent in the AIs for water. Those same determinants of energy expenditure and recommended intake are also applicable to water utilization and balance and this provides an argument for pegging water/fluid intake recommendations to the better studied energy recommendations. The extent to which water intake and requirements are determined by energy intake and expenditure is understudied but in the clinical setting it has long been practice to supply 1 ml per kcal administered by tube to patients unable to take in food or fluids. Factors such as fever or other drivers of increased metabolism affect both energy expenditure and fluid loss and are thus linked in clinical practice. This concept may well deserve consideration in the setting of population intake goals.
Finally, for decades there has been discussion of expressing nutrient requirements per 1000 kcal so that a single number would apply reasonable across the spectrum of age groups. This idea, which has never been adopted by the Institute of Medicine (IOM) and National Academy of Science, may lend itself to an improved expression of water/fluid intake requirements which must replace the AIs eventually. Table 5 presents the IOM water requirements and then develops a ratio of ml/kcal based on them. The European Food Safety Agency refers positively to the possibility of expressing water intake recommendations in ml/kcal as a function of energy requirements. 105 Outliers in the adult male categories which reach ratios as high as 1.5 may well be based on American AI data which are above those in the more moderate and likely more accurate European recommendations.
Exploring the topic of utilizing ml/kcal as the way to examine water intake and water gaps, we take the full set of water intake AIs for each age-gender grouping and examine total intake in Table 6 . They suggest a high level of fluid deficiency. Since a large proportion of fluids in the US are based on caloric beverages and this proportion has changed markedly over the past 30 years, fluid intake increases both the numerator and denominator of this ml/kcal relationship. Nevertheless even using 1 ml/kcal as the AI would leave a gap for all children and adolescents. We then utilize the NHANES physical activity data translated into METS/day to categorize all individuals by physical activity level and thus varying caloric requirements. Using these measures show a fairly large fluid gap, particularly for adult males as well as children.
Water intake and water intake gaps based on US Water Adequate Intake Recommendations (based on utilization of water and physical activity data from NHANES 2005–6.
Note: Recommended water intake for actual activity level is the upper end of the range for moderate and active.
IV. DISCUSSION
This review has pointed out a number of issues related to water, hydration and health. As undoubtedly the most important nutrient and the only one whose absence will be lethal within days, understanding of water measurement and requirements are very important. The effects of water on daily performance and short and long-term health are quite clear. There are few negative effects of water intake and the evidence of positive effects is quite clear from the literature.
Little work has been done to measure total fluid intake systematically and there is no understanding of measurement error and best methods of understanding fluid intake. The most definitive US and European documents on total water requirements as based on these extant intake data. 3 , 105 We feel that absence of validation of methods for water consumption intake levels and patterns represents a major gap. Little exploration of even varying methods of probing to collect better water recall data have been conducted.
Of course, the other half of the issue is the need for understanding total hydration status. We have no acceptable biomarkers of hydration status at the population level. Controversy exists about current knowledge of hydration status among older Americans. 6 , 121 This represents a topic understudied at the population level though certainly scholars are focused on attempting to create biomarkers for measurement of hydration status.
As we have noted, the importance of understanding the role of fluid intake on health has emerged as a more important topic partially because of the shift toward large proportions of fluids coming from caloric beverages. We summarized briefly a related systematic review of the clinical, epidemiological and intervention literature on the effects of added water on health. As a replacement for SSB’s, juice, or whole milk there are clear effects in that energy intake is reduced by about 10–13% of total energy intake. However on these topics, there are only a few longer-term systematic interventions and no published random-controlled longer-term trials. There is very minimal evidence on the effects of just adding water to the diet and of replacing water with diet beverages.
Limitations to this review are many. One certainly is the omission of discussions of the issue of potential differences in the metabolic functioning of different types of beverages 122 . There is little basis at this point for delving into this sparse literature. Another is omission of the potential effects of fructose (from all caloric sweeteners) when consumed in caloric beverages on abdominal fat and subsequently all the metabolic conditions directly linked with this (e.g., diabetes). 123 – 126 We do not review in any detail all the array of biomarkers being considered to measure hydration status as there is just no sense in the field today that there is a measurement that covers more than a very short time period except for 24-hour total urine collection.
We suggest some ways to examine water requirements as a means to encouraging more dialogue on this important topic. Given the importance of water to our health and of caloric beverages to our total energy intake and potential risks of nutrition-related non communicable diseases, understanding both the requirements for water related to energy requirements, and the differential effects of water vs. other caloric beverages remain important outstanding issues.
In the end, this review has attempted to provide some sense of the importance of water to our health, its role in relationship to the rapid increases of obesity and other related diseases, and our gaps in understanding measurement and requirements. Water is essential to our survival and to our civilizations’ and we hope this critical role will sharpen our focus on water in human health.
Acknowledgments
Funding was provided by the Nestlé Waters, Issy-les-Moulineaux, France, 5ROI AGI0436 from the National Institute on Aging Physical Frailty Program, and NIH R01- CA109831 and R01-CA121152. We also wish to thank Ms. Frances L. Dancy for administrative assistance, Mr. Tom Swasey for graphics support, Dr. Melissa Daniels for assistance, and Florence Constant (Nestle’s Water Research) for advice and references.
Contributor Information
Barry M. Popkin, Department of Nutrition, University of North Carolina, Chapel Hill, NC.
Kristen E. D’Anci, Department of Psychology, Tufts University, Medford, MA.
Irwin H. Rosenberg, Nutrition and Neurocognition Laboratory, Jean Mayer USDA Human Nutrition Research Center on Aging at Tufts University, Boston, MA.

The Colorado Sun
Telling stories that matter in a dynamic, evolving state.
Cherish that hamburger. It cost a quarter of the Colorado River, according to researchers.
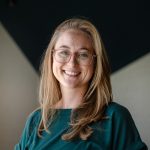
Share this:
- Click to share on Facebook (Opens in new window)
- Click to share on Reddit (Opens in new window)
- Click to share on X (Opens in new window)
- Click to email a link to a friend (Opens in new window)
- Original Reporting
- Subject Specialist
The Trust Project

Next time you take a bite out of a juicy hamburger or chunk of cheese made in the western U.S., say thank you to the Colorado River.
Researchers released the most complete accounting so far of how the river’s water is used . They found that alfalfa — used as feed for beef and dairy cows — sucks up more water than all the cities and industries in the enormous Colorado River Basin.
The researchers incorporated new factors, like water used by nature, Mexico and out-of-basin water transfers, that haven’t been counted before. And with those numbers, the researchers made it clear that water use in the basin needs to drop by 22% to 29% to stabilize historically low reservoirs.
The research, published March 28, comes just in time: Seven state negotiators, 30 tribal nations and federal officials are all weighing how the river’s water will be managed for decades to come.
“Because of these intense negotiations going on over the future management of the Colorado River, we were quite anxious to get the most accurate numbers in front of those negotiators,” said Brian Richter, the study’s lead author and president of Sustainable Waters, a global organization focused on water scarcity challenges.
The U.S. Bureau of Reclamation, which owns and operates massive infrastructure in the Colorado River Basin, is the primary accountant of the river’s water. But past efforts to show how that water is used haven’t taken key factors into account, according to the study, published in the academic journal Communications Earth & Environment.
Reclamation incorporated water sucked up by plants and lost to the atmosphere, but only in riverfront ecosystems in the lower Colorado River. A 2012 report did not include the Gila River basin, which provides most of the water for the Phoenix area.
Other studies haven’t included water use in Mexico, tied to evaporation from reservoirs, or from transfers out of the basin to places including Denver, Fort Collins and Colorado Springs. Even a 2020 study led by Richter did not include all the relevant uses — and that has created uncertainty all around, Richter said.
The oft-cited study estimated that agriculture represented nearly 80% of the water used in the basin, but that study did not include usage by Mexico, the environment, exports out of the basin or through reservoir evaporation. When those uses are taken into account, agriculture represents half the overall use.
“We thought that it was about time for somebody to do an accounting for where all of the water goes,” Richter said. “We really wanted to bring nature into that conversation.”

A new, improved water budget
Richter worked with 11 other researchers from universities around the country to compile what they say is the first complete accounting of water use in the Colorado River Basin.
Their research shows that the Colorado River was overused in 16 of the 21 years during the research period, 2000-2020. Water users overdrafted by 10% on average, driving down the basin’s biggest reservoirs, lakes Mead and Powell, which act like enormous water savings banks.
It may not be a surprise that irrigated agriculture uses the most water, taking up 52% of the water consumed across the 246,000-square-mile basin. But a shocking one-half of that is from just one crop used to feed livestock rather than humans: alfalfa.
Across the basin, alfalfa hay uses more than 5 million acre-feet of Colorado River water — that’s 26% of all the water consumed in the basin. One acre-foot roughly equals the annual water use of two or three households.
In the Upper Basin, irrigated agriculture consumes 2.8 million acre-feet, or nearly half the water used by Colorado, New Mexico, Utah, Wyoming, the environment, reservoirs and water exports out of the basin. Cattle-feed crops, like alfalfa, consume 90% of all water used by Upper Basin agriculture.
That means alfalfa uses more water than cities, commercial users and industries across the entire basin: Collectively, these users consume about 3.5 million acre-feet, or 18%, of the river’s water. The Colorado River provides water to 40 million people across seven Western states, 30 tribal nations and two states in Mexico.
Basinwide alfalfa production also uses more than the water sucked up by riparian ecosystems near rivers and wetlands, which use 19% of the river’s water.
Accounting for nature’s uses is one of the most helpful parts of the study, said Mehdi Nemati, assistant professor of environmental economics and policy at the University of California, Riverside.
“If there is a cutback, alfalfa and hay and pasture — those are the ones to go first. This is just purely looking at direct economic impacts,” said Nemati, who is working on an economic model to estimate the economic impacts of different policy decisions and climate scenarios.
Crops like alfalfa are relatively drought tolerant, always in demand and can be harvested by machine, a more affordable option than human labor. They also act like a buffer in dry years, when farmers can switch to other crops, Nemati said.
“If we don’t have that buffer then who has to go first, right?” he said.
“We’ll be in trouble again”
All of these water users are facing a stress-filled future.
Natural and human consumption amounted to 19.3 million acre-feet on average between 2000 and 2019. Human uses, alone, equaled around 13.6 million acre-feet, according to the study.
The amount of water that is available for human use, in reality, looks more like 13 million acre-feet, Richter said. That gap grows when unused tribal water rights and additional needs are taken into consideration, he said.
The overstressed river basin is already feeling the impacts of climate change. For every 1.8 degree of warming in the Upper Basin, the basin loses 4% to 10% of its water supply, said Peter Goble, a climatologist with the Colorado Climate Center.
“When you consider that there is a very real possibility of warming an additional (3.6 degrees) over current levels, that’s looking at roughly a 10 to 20% reduction in streamflows,” Goble said. “Which is huge when you think about how we’re already dealing with shortages.”
☀️ READ MORE
Environmental groups appeal colorado’s water quality permit for suncor , colorado medicaid program settles lawsuit over lack of mental health care for children, inside the summit county ers that treat thousands of skiing injuries every season.
Based on their comprehensive accounting, the researchers estimated that water users across the basin would need to cut their consumption by 2.4 million acre-feet to 3.4 million acre-feet each year to stabilize reservoir levels. Water users would need to cut an additional 811,000 to 2.4 million acre-feet per year between now and 2050 to account for climate impacts.
The cuts are roughly equal to a historic call in 2022 from the Bureau of Reclamation for states to reduce their use by 2 million acre-feet to 4 million acre-feet.
That means belt-tightening and economic impacts across the basin, Nemati said. Incorporating natural uses was one of the most helpful pieces of the new study. Next, he hopes researchers will model economic impacts based on these numbers — and that public policy will catch up to the data.
“It’s just overcommitted, and we’re not meeting our commitments,” Nemati said. “If we don’t know what is going to the environment, and we go into the negotiations in a year or so … then we can overcommit again. And in a couple of years, we’ll be in trouble again.”
Type of Story: News
Based on facts, either observed and verified directly by the reporter, or reported and verified from knowledgeable sources.
Shannon Mullane Colorado River Reporter
Shannon Mullane writes about the Colorado River Basin and Western water issues for The Colorado Sun. She frequently covers water news related to Western tribes, Western Slope and Colorado with an eye on issues related to resource management,... More by Shannon Mullane

An official website of the United States government
Here's how you know
Official websites use .gov A .gov website belongs to an official government organization in the United States.
Secure .gov websites use HTTPS A lock ( Lock A locked padlock ) or https:// means you’ve safely connected to the .gov website. Share sensitive information only on official, secure websites.
Research Highlights
Quantifying the long-term changes of terrestrial water storage and their driving factors.
Long-term terrestrial water storage (TWS) trends were dissected and quantified using ELM v1 factorial simulations across diverse biomes and climate impacts.
By uncovering the intricate influences of climate, land use, and CO 2 on terrestrial water storage over long-time period, this research enriches our comprehension of global hydrological shifts, informs strategies for water resource resilience, and provides valuable insights for environmental stakeholders and researchers.
Significant drivers of changes in TWS from 1948 to 2012 were quantified, with climate change identified as the dominant factor, particularly affecting temperate regions. Shifts in land use, especially deforestation for agriculture, were also notable influences. The study further revealed complex TWS responses to varying CO 2 levels, with distinct regional disparities. The findings underscore the intricate interplay between human activities and environmental factors in shaping global hydrological patterns, signaling critical implications for future water resource management and climate adaptation strategies.
Quantifying the long-term changes of terrestrial water storage and their driving factors Shi, Xiaoying, Yaoping Wang, Jiafu Mao, Peter Thornton, Daniel Ricciuto, Forrest M. Hoffman, and Yuefeng Hao . 2024 . “Quantifying The Long-Term Changes Of Terrestrial Water Storage And Their Driving Factors” . Journal Of Hydrology 635. doi:10.1016/j.jhydrol.2024.131096.
Recent Headlines
- ESF Traditions Have Deep Roots
- SUNY ESF Named an Adobe Creative Campus
- ESF Ph.D. Student Wins American Chemical Society Award
- SUNY ESF Launches New Safety App
Submit a News Story
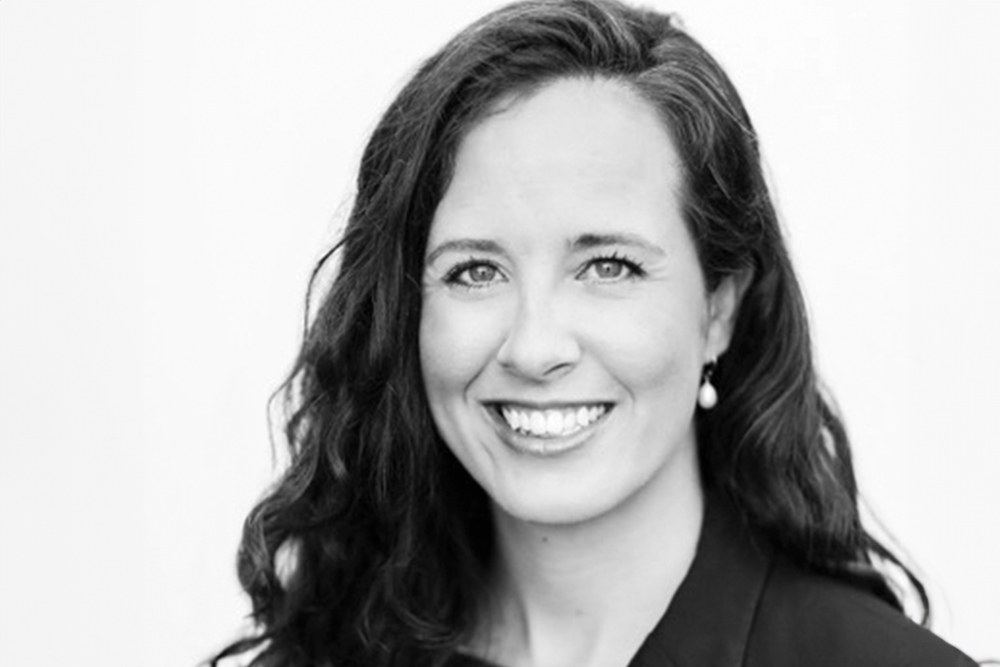
Dr. Melissa Rohde
SUNY ESF Leads Groundbreaking Research in Groundwater’s Role in Ecosystem Sustainability
Syracuse, N.Y. – April 2, 2024 – Until now, groundwater – a critical water resource around the globe, especially in dry regions – has been largely unstudied in its importance and role in sustaining ecosystems. A new groundbreaking research effort led by the SUNY College of Environmental Science and Forestry (ESF) in partnership with University of California Santa Barbara (UCSB), Cardiff University, and Desert Research Institute (DRI) examines the relationship between groundwater and ecosystems across California. Their innovative findings are featured in Nature Water .
Led by Dr. Melissa Rohde, who completed the study as part of her doctoral research in Dr. John Stella’s Riparian Echohydrology Lab at ESF, the team used satellite imagery and groundwater monitoring data to identify thresholds of groundwater depth and seasonal change that can support sensitive ecosystems throughout California under the state’s Sustainable Groundwater Management Act framework.
“A vast majority of our planet’s freshwater is groundwater, but we don’t acknowledge or manage it sustainably, resulting in serious consequences for humans and natural ecosystems,” said Dr. Rohde, now Principal at Rohde Environmental Consulting, LLC. “Groundwater is critical for many ecosystems, but their water requirements are rarely accounted for by water agencies and conservationists. To reconcile that, our study provides a simple and practical approach to detect ecological thresholds and targets that can be used by practitioners to allocate and manage water resources.”
Utilizing 38 years of Landsat satellite images (1985-2022) and statewide groundwater well data, the study examined impacts on key plant communities. A major challenge was to develop standardized metrics that can be applied across diverse ecosystems with site-specific water conditions. The team applied a common data transformation method in a new way to identify thresholds of vegetation greenness and groundwater depth over time that can determine groundwater needs for ecosystems, helping to inform decisions about water use and planning.
“Groundwater-dependent ecosystems such as wetlands, floodplains, and riparian zones have a very outsized importance on biodiversity. Upwards of 80 to 90 percent of species in a general region may be dependent on these ecosystems in some form or another,” said Dr. Stella, study co-author and Vice President for Research at ESF. “We applied a simple statistical approach to very large data sets to identify warning signs and conservation targets for a great diversity of ecosystem types.”
The vast geographic scope and long timeline covered by the study, allowed the team to evaluate how large-scale systems respond to major climate shocks such as the historical California drought that occurred from 2012—2016, as well as where individual groundwater-dependent ecosystems can serve as resilient drought refugia.
“This type of study, covering the entire state of California over close to 40 years, has really only become possible in the past few years and shows the promise for similar studies over a much larger geographic area using the approach pioneered by Dr. Rohde,” remarked co-author, Dr. Dar Roberts from UCSB.
It was discovered that during drought events, groundwater-dependent vegetation that maintains a connection to groundwater can serve as critical drought refugia for associated species, such as riparian birds or fish. However, when groundwater levels deepen beyond plants’ rooting zones during drought, these safe havens can be lost.
"A key takeaway from this study is that we can use what we know about how deep the roots of different types of plants tend to be to approximate what groundwater levels are needed to maintain ecosystem health,” said co-author Dr. Christine Albano from DRI. “We found that vegetation was healthier where groundwater levels were within about 1 meter of maximum root depth, as compared to where groundwater was deeper."
The research team is hopeful that their approach and findings can help inform water management decisions in California and beyond.
"This study arms groundwater managers with an intuitive, site-specific measure that can provide a data-driven foundation to guide water allocation and ecosystem restoration efforts," said co-author and professor Kelly Caylor from UCSB.
“Globally, there are increasing efforts to manage groundwater resources for multiple purposes, not only to support drinking water needs or high-value agriculture. Our work provides a sound basis on which to develop clear guidelines for how to manage groundwater to support a wide range of needs within drainage basins in California and beyond,” said co-author Prof. Michael Singer from Cardiff University.
About SUNY ESF
The SUNY College of Environmental Science and Forestry (ESF) is dedicated to the study of the environment, developing renewable technologies, and building a sustainable and resilient future through design, policy, and management of the environment and natural resources. Members of the College community share a passion for protecting the health of the planet and a deep commitment to the rigorous application of science to improve the way humans interact with the world. The College offers academic programs ranging from the associate of applied science to the Doctor of Philosophy. ESF students live, study and do research on the main campus in Syracuse, N.Y., and on 25,000 acres of field stations in a variety of ecosystems across the state.
Water scarcity in the Kingdom of Saudi Arabia
- Review Article
- Published: 08 April 2024
Cite this article
- Faiza I. A. Abdella 1 ,
- Walaa I. El-Sofany 1 , 2 &
- Dorsaf Mansour 1
Saudi Arabia (SA) is one of the world’s arid, most water-scarce nations without permanent water resources. The purpose of this article is to provide a comprehensive overview of Saudi Arabia’s water resources availability and reliability in terms of water supply, demand, and the major challenges that water faces. Saudi has an annual water supply of roughly 89.5 m 3 per person as consumption is rising in parallel with the country’s rapid population growth and development. SA produces the most desalinated seawater in the world, accounting for 22% of worldwide consumption. Due to changes in agricultural demand, Saudi Arabia’s overall water needs in 2020 were 15.98 BCM. Apart from agricultural use, the food industry utilizes up to 80% of freshwater supplies, with only around 20% of rain recharging the aquifer, meaning that the region will still be water-stressed by 2025. In addition to wastewater reuse, water expenses should be split between private investors and the government, and water losses in cities should be collected and recycled. Water development projects must also be safeguarded and have long-term viability for the community’s future and well-being. Despite previous conservation efforts (public awareness campaigns, television and other public media messages, drip irrigation, and so on), more work is required, including improving water resource infrastructure, implementing environmental use of friendly technologies, and increasing economic feasibility, social acceptability, and management in light of the Sustainable Development Goals (SDG).
This is a preview of subscription content, log in via an institution to check access.
Access this article
Price includes VAT (Russian Federation)
Instant access to the full article PDF.
Rent this article via DeepDyve
Institutional subscriptions
Data availability
This article is derived from public resources and made available with the article.
Abderrahman WA (2000b) Water demand management and Islamic principles: a case study. Water Resour Dev 16(4):465–473
Article Google Scholar
Abderrahman, W.A., 2000a. Water demand management in Saudi Arabia. In: Farukui, N.I., Biswas, A.K., Bino, M.J. (Eds.), Water Management in Islam. The International Development Research Centre (IDRC), pp. 68–78.
Alcamo, J., Döll, P., Kaspar, F., Siebert, S. Global change and global scenarios of water use and availability: an application of WaterGAP 1.0; University of Kassel: Kassel, Germany, 1997.
Al-Farra, T. Water security in the Gulf region. Gulf cooperation council’s challenges and prospects. Proceedings of the 11 th Kovacs Colloquium, Paris, France, 2015; 101pages. IAHS Publisher.
Ali Mahmoud MS, Abdallh SM (2013) Water-demand management in the Kingdom of Saudi Arabia for enhancement environment. Comp Eng Intel Syst 4:26–49
Google Scholar
Al-Ibrahim AA (1990) Water use in Saudi Arabia: problems and policy implications. J Water Resour Plan Manag 116(3):375–388
Alkhudhiri A, Darwish NB, Hilal N (2019) Analytical and forecasting study for wastewater treatment and water resources in Saudi Arabia. J Water Proc Eng 32(100915):2021. https://doi.org/10.1016/j.jwpe.2019.100915
Alkolibi FM (2002) Possible effects of global warming on agriculture and water resources in Saudi Arabia: impacts and responses. Clim Change 54:225–245
Almazroui M, Abid MA, Athar H, Islam MN, Ehsan MA (2013) Inter-annual variability of rainfall over the Arabian Peninsula using the IPCC AR4 Global Climate Models. Int J Climatol 33(10):2328–2340
Alobireed, A. Global Water Desalination: a comparison between Saudi Arabia and the United States of America. 2021; 38 pages. Master dissertation, University of Pittsburgh, Pennsylvania, United States. http://d-scholarship.pitt.edu/id/eprint/40982 . Accessed at 6 March, 2021.
Alrwis KN, Ghanem AM, Alnashwan OS, Al Duwais AM, Alaagib SB, Aldawdahi NM (2021) Measuring the impact of water scarcity on agricultural economic development in Saudi Arabia. Saudi J Biol Sci 28(1):191–195
Al-Suhaimy U. Saudi Arabia: the desalination nation. Asharq Al-Awsat. July 2, 2013. Available at: http://www.aawsat.net/2013/07/article .
Al-Zahrani KH, Baig MB, Straquadine GS. Food waste in the Kingdom of Saudi Arabia: implications for extension education, in food security and natural resource management, Climate Change, Springer, Cham, (2019); 73–101.
AQUASTAT. Saudi Arabia. Food and Agriculture Organization of the United Nations web site. Available at: http://www.fao.org/nr/water/aquastat/countries_regions/sau/index.stm . Accessed August 17, 2015.
Assaf, H. Water resources and climate change, in water sustainable management of a scarce resource, Arab Forum for Environment and Development, Beirut, Lebanon, (2010); 25–38.
Ayres, R.M., Mara, D.D., 1996. Analysis of wastewater for use in agriculture: a laboratory manual of parasitological and bacteriological techniques. World Health Organization (WHO), Geneva, Switzerland.
Chang, A.C., Pan, G., Page, A.L., Asano, T., 2002. Developing human health-related chemical guidelines for reclaimed water and sewage sludge applications in agriculture. Prepared for World Health, Organization, 2002.
Chowdhury S, Al-Zahrani M (2015a) Characterizing water resources and trends of sector wise water consumptions in Saudi Arabia. J King Saud Univ Eng Sci 27(1):68–82
Chowdhury, S., Al-Zahrani, M., 2012. Implications of climate change on water resources in Saudi Arabia. The Arabian J. Science and Engineering (AJSE), in press. https://doi.org/10.1007/s13369-013-0565-6 .
Chowdhury, S., Champagne, P., 2006. Use of treated wastewater: evaluation of wastewater minimization strategies using fuzzy technique. In: Proceedings of the Conference on Advanced Technology in the Environmental Field (ATEF), February 6–8, 2006. Spain.
CIA, 2011. CIA Fact Book: Available at: < https://www.cia.gov/library/publications/the-world-factbook/ > (accessed 20.09.11).
Damkjaer S, Taylor R (2017) The measurement of water scarcity: defining a meaningful indicator. Ambio 46(5):513–531
Davis J (2005) Private-sector participation in the water and sanitation sector. Annu Rev Environ Resour 30:145–183
DeNicola E, Aburizaiza OS, Siddique A, Khwaja H, Carpenter DO (2015a) Climate change and water scarcity: the case of Saudi Arabia. Ann Glob Health 81(3):342–353
Dosi C, Easter KW (2002) Water scarcity: institutional change, water markets, and privatization in economic studies on food, agriculture, and the environment. Springer, Boston, MA, pp 91–115
Drewes JE, Patricio RC, Amy GL (2012a) Water reuse in the Kingdom of Saudi Arabia–status, prospects and research needs. Water Sci Technol: Water Supply 12(6):926–936
Elhadj, E., 2004. Household water and sanitation services in Saudi Arabia: an analysis of economic, political and ecological issues. SOAS Water Research Group, Occasional Paper 56.
Evenett SJ (2019) Protectionism, state discrimination, and international business since the onset of the global financial crisis. J Int Bus Policy 2(1):9–36
Falkenmark M, Lundqvist J, Widstrand C (1989) Macro-scale water scarcity requires micro-scale approaches-aspects of vulnerability in semi-arid development. Nat Res Forum 13:258–267
Article CAS Google Scholar
FAO (Food and Agriculture Organization of the United Nations), 2003. Review of World Water Resources by Country, 2003. Water Report No. 23; FAO: Rome, Italy.
FAO (Food and Agriculture Organization), 1998. Proceedings of the Second Expert Consultation on National Water Policy Reform in the Near East, Cairo, Egypt, 24–25 November 1997.
FAO (Food and Agriculture Organization), 2009. Irrigation in the Middle East region in figures. Food and Agriculture Organization of the United Nations. FAO Water Reports 34, Rome.
Frenken K (2009) Irrigation in the Middle East region in figures AQUASTAT Survey-2008. Water Rep 34:355–375 ( http://www.fao.org/3/a-i0936e.pdf )
GAS, General Authority of Statistics, 2019. Statistical indicators for sustainable development goals, Statistical report on the current status 2019. https://www.stats.gov.sa/sites/default/files/Pdf . Accessed at 24 October, 2021
GAS, General Authority of Statistics, 2020. Quantity of water used and produced from non-conventional sources in Saudi Arabia in 2010–2018. https://www.stats.gov.sa/sites/default/files/quantity_of_water_used_and_produced_from_non_-_conventional_sources_in_saudi_arabia_in_2010_-_2018_0 . pdf. Accessed at 24 December, 2021
Ghanim AA (2019) Water resources crisis in Saudi Arabia, challenges and possible management options: an analytic review. Int J Environ Ecol Eng 13(2):51–56
Gleick PH (2000) A look at twenty-first century water resources development. Water Int 25(1):127–138
GOSA, Government of Saudi Arabia, 2016. Vision 2030. http// www.vision2030.gov.sa/en . Accessed at 25 December, 2021.
Haider H, Sadiq R, Tesfamariam S (2014) Performance indicators for small-and medium-sized water supply systems: a review. Environ Rev 22(1):1–40
Halverson, N. What California can learn from Saudi Arabia’s water mystery. Reveal News. April 22, 2015.
Heal the Bay. (2023). Ocean-water desalination: a solution or a problem? Available at: https://healthebay.org/sites/default/files/Desalination%20FAQ%20Sheet_final.pdf .
Jones E, Qadir M, van Vliet MTH, Smakhtin V, Kang S (2019) The state of desalination and brine production: a global outlook. Sci Total Environ 657(2019):1343–1356
Kingdom of Saudi Arabia (KSA) populations, 2020. Urban versus rural from 1955 to 2020. https://www.worldometers.info/demographics/saudi-arabia-emographics/ . Accessed at 14 October, 2020.
Lippman TW. Biggest Mideast crisis: growing water scarcity. Business Mirror. May 24, 2014. Available at: http://www.ipsnews.net/2014/05/biggest-mideast-crisis-probably-dontknow-enough/ . Accessed August 17, 2015.
MAW (Ministry of Agriculture and Water), 1982. Census of agriculture according to farm size, 1981–82. MAW, Riyadh.
MAW (Ministry of Agriculture and Water), 1984. Water Atlas of Saudi Arabia. Riyadh, MAW.
MAW (Ministry of Agriculture and Water), 1992. Agricultural Statistical Year Book. Vol. 7, Ministry of Agriculture and Water, Riyadh, Department of Economic Studies and Statistics.
Mays LW (2011) Water Resources Engineering, 2nd edn. John Wiley and Sons Inc, NJ, USA
MEWA, Ministry of Environment, Water and Agriculture. Statistical Year Book; Ministry of Environment, Water, and Agriculture. 2019; 57–96. Riyadh, Saudi Arabia.
MOEP (The Ministry of Economy and Planning), 2010. The Ninth Development Plan (2010–2014). The Kingdom of Saudi Arabia.
Molden D. Water for food water for life: a comprehensive assessment of water management in agriculture. 2013; 664 pages. Routledge Press.
MOP (Ministry of Planning), 1985. Fourth Development Plan: 1985–1990. MOP, Riyadh.
MOWE (Ministry of Water and Electricity), 2009. The Ministry of Water and Electricity Annual Report 2009. Riyadh, Saudi Arabia.
Napoli, C., Wise, B., Wogan, D. and Yaseen, L. (2016). Policy options for reducing water for agriculture in Saudi Arabia. Available at: file:///C:/Users/IEC/Downloads/KS-1630-DP024A-Policy-Options-for-Reducing-Water-for-Agriculture-in-SA.pdf.
Ng SW, Zaghloul S, Ali HI, Harrison G, Popkin BM (2011) The prevalence and trends of overweight, obesity and nutrition-related non-communicable diseases in the Arabian Gulf States. Obes Rev 12:1–13
Ouda OK (2016) Treated wastewater use in Saudi Arabia: challenges and initiatives. Int J Water Resour Dev 32(5):799–809
Panagopoulos A, Haralambous KJ (2020) Environmental impacts of desalination and brine treatment-challenges and mitigation measures. Mar Pollut Bull 161:111773
Pearce, F. When the rivers run dry, fully revised and updated edition: Water-the defining crisis of the twenty-first century, (2018). 311pp. Beacon Press.
Pereira LS, Cordery I, Iacovides I. Coping with water scarcity: addressing the challenges. 2009; 7–24. Springer Science and Business Media.
Postel SL. Securing water for people, crops, and ecosystems: new mindset and new priorities, in Natural Resources Forum. Oxford, UK, (2003); 27(2): 89–98). Blackwell Publishing Ltd.
Pryor FL (2007) Water stress and water wars. Econ Peace Secur J 2(2):6
Qadir M, Sharma BR, Bruggeman A, Choukr-Allah R, Karajeh F (2007) Non-conventional water resources and opportunities for water augmentation to achieve food security in water scarce countries. Agric Water Manag 87(1):2–22
Qadir, M and Smakhtin, V. (2021). Five unusual technologies for harvesting water in dry areas. Available at: https://theconversation.com/five-unusual-technologies-for-harvesting-water-in-dry-areas-154031 .
Samad NA, Bruno VL (2013) The urgency of preserving water resources. Enviro News 2013(21):3–6
Schilling J, Hertig E, Tramblay Y, Scheffran J (2020) Climate change vulnerability, water resources and social implications in North Africa. Reg Environ Change 20(1):1–12
Sojobi AO, Zayed T (2022) Impact of sewer overflow on public health: a comprehensive scientometric analysis and systematic review. Environ Res 203:111609
Sowers J, Vengosh A, Weinthal E (2011a) Climate change, water resources, and the politics of adaptation in the Middle East and North Africa. Clim Change 104(3):599–627
SSYB (Saudi Statistical Year Book), 2008. Water Consumption and Number of Subscribers. SSYB (Chapter 8).
Staff writer. Saudi water desalination industry posts highest global annual growth. Saudi Gazette. May 7, 2014. Available at: http://www.saudigazette.com.sa/index.cfm?method¼home.regcon&contentid¼20140507204347 . Accessed August 17, 2015.
Population Stat, 2003. Historical demographical data for Saudi Arabia. Available at: < http://www.populstat.info/Asia/saudiarc.htm >.
SWCC, 2011. General organization of water desalination. Available at: < http://www.swcc.gov.sa/default.asp >.
SWCC, Saline Water Conversion Corporation, 2019. Annual Report, 2019. https://www.swcc.gov.sa/Arabic/MediaCenter/SWCCPublications/Publication files/Annual_Report_2019579972DE-2CB9–4E02–91F3–0DDB5D025391.Pdf. Accessed at 8 March, 2021.
Tarawneh QY, Chowdhury S (2018) Trends of climate change in Saudi Arabia: implications on water resources. Climate 6(1):8
UN (United Nations), 2020. Department of Economic and Social Affairs, Statistics Division. Sustainable Development Goal Indicators, 2020. https://unstats.un.org/sdgs/report/2020/goal-06/ . Accessed on 15 March 2021.
UNDESA, United Nations Department of Economic and Social Affairs. Population Division. World Urbanization Prospects: The 2018 Revision (ST/ESA/SER. A/420), 2019; New York: United Nations.
Ursula, J.B., Peasey, A., 2002. Critical review of epidemiological evidence of the health effects of wastewater and excreta uses in agriculture. London School of Hygiene and Tropical Medicine.
USEPA, 2009. US Environmental Protection Agency. Review draft on control and mitigation of drinking water losses in distribution system. Office of Water (OW/OGWDW/DWPD), EPA MC-4606M. EPA 816-D-09–001, Washington DC.
World Bank Group (WB), 2021. Catalog Sources: World Development Indicators, data source: Food and Agriculture Organization, AQUASTAT data, 2021. http://data.worldbank.org/indicator . Accessed at 19 October, 2021.
Zekri S, Al-Maamari A. An overview of the water sector in MENA Region. Water Policies in MENA Countries, (2020); 1–17.
Zuhair, A., Nouh, M., El-Sayed, M., 1999. Flood harvesting in selected Arab States. Final Report, Institute of Water Resources.
Download references
Author information
Authors and affiliations.
Department of Chemistry, Faculty of Science, University of Hail, Hail, Saudi Arabia
Faiza I. A. Abdella, Walaa I. El-Sofany & Dorsaf Mansour
Photochemistry Department, Chemical Industries Research Institute, National Research Centre, Scopus Affiliation ID 60014618, 33 EL Buhouth St., Dokki, 12622, Giza, Egypt
Walaa I. El-Sofany
You can also search for this author in PubMed Google Scholar
Contributions
All of the authors (Faiza I.A. Abdella, Walaa I. El-Sofany, Dorsaf Mansour) contributed equally to data collection, drafting, processing, writing, editing, and reviewing the article.
Corresponding author
Correspondence to Faiza I. A. Abdella .
Ethics declarations
Ethical approval.
This article reviews free data from Web of Science, Google Scholar, Google Webpage, and other websites that include replicable, up-to-date methods without the need for ethical approval aside from acknowledgments of authors and/or resources regarding Saudi Arabia’s water resources availability and reliability in terms of water supply, demand, and its major challenges.
Consent to participate
We (Faiza I.A. Abdella, Walaa I. El-Sofany, Dorsaf Mansour) voluntarily agree to take part in this study. We understand we will receive and sign a copy of this consent form.
Consent for publication
The authors (Faiza I.A. Abdella, Walaa I. El-Sofany, Dorsaf Mansour) declare that all of the works are original and that this paper has not been published in any other journals.
Competing interests
The authors declare no competing interests.
Additional information
Responsible Editor: Philippe Garrigues
Publisher's Note
Springer Nature remains neutral with regard to jurisdictional claims in published maps and institutional affiliations.
Rights and permissions
Springer Nature or its licensor (e.g. a society or other partner) holds exclusive rights to this article under a publishing agreement with the author(s) or other rightsholder(s); author self-archiving of the accepted manuscript version of this article is solely governed by the terms of such publishing agreement and applicable law.
Reprints and permissions
About this article
Abdella, F.I.A., El-Sofany, W.I. & Mansour, D. Water scarcity in the Kingdom of Saudi Arabia. Environ Sci Pollut Res (2024). https://doi.org/10.1007/s11356-024-33024-3
Download citation
Received : 14 August 2022
Accepted : 17 March 2024
Published : 08 April 2024
DOI : https://doi.org/10.1007/s11356-024-33024-3
Share this article
Anyone you share the following link with will be able to read this content:
Sorry, a shareable link is not currently available for this article.
Provided by the Springer Nature SharedIt content-sharing initiative
- Saudi Arabia (SA)
- Water scarcity
- Water supply and demand
- Water challenges
- Conservation efforts
- Find a journal
- Publish with us
- Track your research

Site Search
- About ARPA-E
- Team Directory
- ARPA-E History
- Annual Reports
- Budget Requests
- Apply For Funding
- Authorization
- View Active Programs
- Search Our Programs
- Search Individual Projects
- Interactive Project Map
- Exploratory Topics
- The SCALEUP Program
- OPEN Programs
- Project Guidance
- ARPA-E Technology-to-Market
- Technology Commercialization
- External Engagement Model
- Investor Updates
- ARPA-E News & Media
- Press Releases
- ARPA-E Disruptors
- Publications
- ARPA-E Events
- Energy Innovation Summit
- Careers at ARPA-E
- Job Opportunities
- Life at ARPA-E
- ARPA-E FAQs
- General Questions
- Current Funding Opportunities
- Closed Funding Opportunities
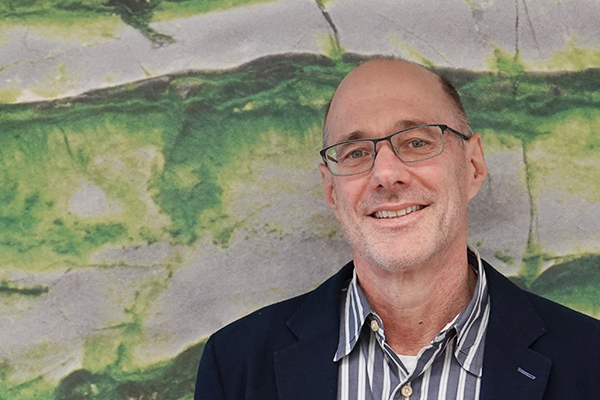
Meet the Program Director: Dr. Charles Werth
Dr. Charles Werth is a new ARPA-E Program Director. His research prior to joining ARPA-E focused on the intersection of mass transport, fluid flow, interfacial and geo-chemistry, redox reactions, microbial physiology, and material science for the development of sustainable water treatment, groundwater remediation, and geological carbon sequestration technologies.
What brought you to ARPA-E?
I came to ARPA-E because of the opportunity to help advance early-stage technologies that address water-energy challenges. I’ve worked on research related to water supply and treatment for most of my career, and I have a strong interest in how water impacts energy use and vice versa. As an academic, however, I was more focused on fundamental research than technology development and commercialization. ARPA-E presents an entirely new challenge and opportunity to think about moving research to the next level, and to have a more direct impact on water-energy challenges. I think addressing these challenges is particularly important because many cities in the U.S. are struggling to provide clean water economically. Also, we expect energy demands for water supply and treatment to grow with increasing water scarcity as we treat and use increasingly compromised or distant water sources. Additionally, I came to ARPA-E to learn about and contribute to the development of new technologies for other energy challenges, including carbon sequestration and subsurface resource recovery.
Tell us about your background.
I received my graduate training in environmental engineering, focusing on physical and chemical processes that affect the reactive transport of pollutants in groundwater. After graduating, I entered academia, and have been a professor for the past 27 years, first at the University of Illinois at Urbana-Champaign and since 2014 at the University of Texas at Austin. My interests have expanded since graduate school, and I’ve guided interdisciplinary research projects that focus on sustainable water treatment, groundwater remediation, and geological carbon sequestration. Most of my research has been funded by federal agencies, including the DOE, DOD, NSF, EPA, and USGS. I’ve advised 27 Ph.D. students who graduated and went on to careers in academia, industry, and consulting. I’ve served on a number of review and advisory panels. Among them, I served on the U.S. EPA Science Advisory Board from 2014-2017, where I enjoyed contributing to addressing environmental challenges at the intersection of science and policy. I also served as a Chief Editor for the Journal of Contaminant Hydrology from 2014-2023, where I enjoyed helping to expand the journal scope and broadening my exposure to great research.
How does your past work drive your current work supporting ARPA-E’s mission of developing entirely new ways to generate, store, and use energy?
In my prior work on water and wastewater treatment, I learned that energy represents a large fraction of treatment costs, and that wastewater contains many valuable materials that we remove during treatment for disposal. This drives my efforts to develop a new program area on innovative technologies to recover high energy-value materials from wastewater. In my prior work on geological carbon sequestration, I learned about mechanisms that affect the flow, transport, and reaction of various fluids in deep subsurface formations, including CO 2 , hydrocarbons, and hydrogen. This motivates and informs my efforts to contribute to programs in carbon capture, storage, and subsurface resource recovery.
What’s it been like to work at ARPA-E now that you’ve got a few months under your belt to reflect?
Working at ARPA-E has been great. My colleagues are engaged, smart, and supportive. They have very different backgrounds, and I’m learning about so many new energy challenges and technologies from them. To build my first ARPA-E research program, I’ve been engaging with lots of different people. This includes engineers and scientists, as well as CEOs, CTOs, and Technology-to-Market experts. These engagements have broadened my perspective and knowledge of energy challenges and possible solutions and are helping me better understand the bottlenecks that can derail technology commercialization.
In what innovative ways would you like to explore carbon sequestration technologies and mitigating the environmental impacts of energy production?
Carbon sequestration technologies are advancing at an amazing pace. Last year, the DOE announced $1.2 billion for development of two commercial-scale direct air capture facilities. Despite these advancements, carbon sequestration technologies are still in their infancy. We need early-stage applied research to discover new materials and material properties that will enable us to more selectively sequester CO 2 from air and other mixed gas streams, and we need new and less energy-intensive processes to permanently store CO 2 . I look forward to working with my colleagues at ARPA-E to identify and support projects that target the most promising materials and processes to address these fundamental challenges.
What new program areas or technical whitespaces are you interested in exploring?
I’ve been working in water my entire adult life and am interested in substantially decreasing energy demands and carbon emissions associated with water supply and treatment. As I mentioned earlier, my first efforts at ARPA-E are focused on developing a new program on high energy-value materials recovery from wastewater, where wastewater is broadly defined to include municipal, animal, industry, and reverse osmosis concentrate sources. Two other areas I’m interested in are electrification of chemical oxidation technologies in water and wastewater treatment and using artificial intelligence for long-range water planning. The latter area would have broad implications for how municipalities and cities plan for future water resources and treatment, and could have immense energy implications when cities have to choose between pumping a cleaner source water from further away or treating a more compromised source water closer to home.
What do you hope to accomplish during your tenure at ARPA-E?
I hope to lead development of at least a few new programs at the water-energy nexus. I hope to oversee other diverse energy recovery and carbon emissions reduction projects, and to help guide applied research efforts that lead to new cutting-edge technologies and commercialization. I hope to make friends, have fun, and learn what makes or breaks commercialization efforts of new technologies across the energy space.

Report: 90% of CO River irrigation water used to feed cattle

As Colorado and other states grapple with shrinking Colorado River water levels - new research pinpoints how much water is being diverted to feed cattle, to sprawling desert cities, and the river's 40 million other stakeholders.
The stakes are high in a time of persistent and widespread drought.
Brian Richter - president of Sustainable Waters - said if Upper Basin states can't deliver the volume of water required under a century-old agreement, Lower Basin states could force the issue with what's known as a compact call.
"The likely result would be that the Upper Basin states (including Colorado) would be forced to use less water," said Richter, "so that more water could be flowing into Lake Powell and downstream into the Lower Basin."
Researchers found that in Upper Basin states, cattle-feed crops soak up 90% of all irrigation water - which is three times the amount that goes to all cities, towns, commercial and industrial uses combined.
Just 19% of the Colorado River feeds the wetlands and riparian areas wildlife depend on.
Richter noted that cities along Colorado's Front Range and in Utah are at risk because they have very low priority for accessing water under the 1922 Colorado River Compact.
Despite calls for closing off spigots used exclusively for cattle feed, Richter said blaming any single user is counter-productive.
"Farmers and ranchers are growing the things that people want, and are willing to pay a necessary price for," said Richter. "So they are just responding to consumer demands."
He said he believes the new data could be an important tool for Colorado River stakeholders as they work to build a long term plan to bring the total use of water back in balance with what nature provides.
Richter said right now, water use is at least 10% to 15% over that limit.
"We need a long range plan that says how much water do we want to use in the cities? How much water do we want to use on the irrigated farms? How much are the industries going to need?" said Richter. "And until we do that long range plan, we are just going to be reacting to these water shortages on a year-by-year basis."
Fight coronavirus (COVID-19) transmission at home
Used properly, most common household disinfectants will kill the virus that causes COVID-19.
Getting your COVID-19 vaccine is the best way to lower your risk of serious illness. Getting the vaccine also prevents the spread of the COVID-19 virus.
In addition to the vaccine there are other actions you can take to keep this coronavirus from spreading in your home. Wash your hands well and often to lower your risk of infection. Improving air flow in your home and filtering the air can help. And for the coronavirus and other germs, clean those high-touch surfaces in your home and disinfect them when needed.
What's the difference between cleaning and disinfecting?
Cleaning removes most germs and dust or dirt. Disinfecting kills most germs. If someone in your home has COVID-19, clean first, then disinfect.
How is COVID-19 spread?
The virus that causes COVID-19 spreads mainly from person to person. It can spread from people who are infected but have no symptoms. When people with COVID-19 cough, sneeze, breathe, sing or talk, the virus carried on their breath can land on the faces of people nearby. The virus spreads when other people breathe in infected droplets or when the droplets land in their eyes, noses or mouths.
The COVID-19 virus also can spread if people touch their eyes, noses or mouths after touching a surface with the virus on it. Without cleaning and disinfection, the COVID-19 virus may stay on surfaces from hours to days. But the risk of COVID-19 through contact with infected surfaces seems low.
How can I clean and disinfect my home?
You can lower the risk of spreading of the COVID-19 virus by focusing on surfaces that are touched often. Examples are tables, doorknobs, light switches, handles, counters, desks, toilets, faucets and sinks. Clean these things with soap and water or with a product made for the specific surface. Follow the instructions on the product label.
Most often, cleaning is enough to lower the risk. Clean more often if someone in your home is at higher risk of severe illness from COVID-19.
If someone who is sick with COVID-19 lives with you or has been in your home within the last 24 hours, disinfect surfaces right after cleaning them. Disinfecting helps kill germs that are left.
Disinfecting uses strong chemicals.
Read product labels before use and follow instructions carefully. Many disinfectants need to stay on surfaces for some time to work. This is called the contact time, and the label will tell you that length of time.
Put on gloves before disinfecting. Disposable gloves are best because you can throw them away when you're done. If you have only gloves that you reuse, don't use them for anything else. Wash your hands well with soap and water for 20 seconds right after cleaning and disinfecting.
Keep doors or windows open and use a fan to help increase air flow while disinfecting your home.
What disinfectants kill COVID-19?
The U.S. Environmental Protection Agency (EPA) has a list of disinfectants for use against COVID-19. Look for products with active ingredients such as ethanol, hydrogen peroxide or quaternary ammonium. In the U.S., check labels for EPA registration numbers.
Does bleach work against COVID-19?
Yes. You can make a disinfecting solution by mixing 4 teaspoons (about 20 milliliters) of household bleach and 1 quart (a bit less than 1 liter) of water.
Read and follow instructions on your bottle of bleach. It's also important to wear gloves and make sure there's good airflow in the room. Don't mix bleach with ammonia or any other cleanser because mixing can cause toxic fumes.
How can I disinfect phones and other electronics?
Cellphones are high-touch devices that can carry COVID-19 germs. Follow makers' advice for cleaning and disinfecting them and other electronics. You also may consider covering your phone or shared electronics, such as a keyboard, with a product that can be easily disinfected.
Protect yourself every day
As you touch people, surfaces and objects throughout the day, you get germs on your hands. You can infect yourself with these germs by touching your eyes, nose or mouth.
To protect yourself, wash your hands often with soap and water for at least 20 seconds. If there's no soap and water, use an alcohol-based hand sanitizer with at least 60% alcohol.
- When and how to clean and disinfect your home. Centers for Disease Control and Prevention. https://www.cdc.gov/hygiene/cleaning/cleaning-your-home.html. Accessed Feb. 28, 2024.
- McIntosh K. COVID-19: Epidemiology, virology, and prevention. https://www.uptodate.com/contents/search. Accessed Feb. 28, 2024.
- Smith BA. COVID-19: Infection prevention for persons with SARS-CoV-2 infection. https://www.uptodate.com/contents/search. Accessed Feb. 28, 2024.
- COVID-19: How to protect yourself and others. Centers for Disease Control and Prevention. https://www.cdc.gov/coronavirus/2019-ncov/prevent-getting-sick/prevention.html. Accessed Feb. 28, 2024.
- Cleaning and disinfecting: Best practices during the COVID-19 pandemic. Environmental Protection Agency. https://www.epa.gov/coronavirus/cleaning-and-disinfecting-best-practices-during-covid-19-pandemic. Accessed Feb. 28, 2024.
- List N advanced search page: Disinfectants for coronavirus (COVID-19). https://www.epa.gov/coronavirus/list-n-advanced-search-page-disinfectants-coronavirus-covid-19. Accessed Feb. 28, 2024.
Products and Services
- A Book: Endemic - A Post-Pandemic Playbook
- Begin Exploring Women's Health Solutions at Mayo Clinic Store
- A Book: Future Care
- Antibiotics: Are you misusing them?
- COVID-19 and vitamin D
- Convalescent plasma therapy
- Coronavirus disease 2019 (COVID-19)
- COVID-19: How can I protect myself?
- Herd immunity and coronavirus
- COVID-19 and pets
- COVID-19 and your mental health
- COVID-19 antibody testing
- COVID-19, cold, allergies and the flu
- COVID-19 drugs: Are there any that work?
- Long-term effects of COVID-19
- COVID-19 tests
- COVID-19 in babies and children
- Coronavirus infection by race
- COVID-19 travel advice
- COVID-19 vaccine: Should I reschedule my mammogram?
- COVID-19 vaccines for kids: What you need to know
- COVID-19 vaccines
- COVID-19 variant
- COVID-19 vs. flu: Similarities and differences
- COVID-19: Who's at higher risk of serious symptoms?
- Debunking coronavirus myths
- Different COVID-19 vaccines
- Extracorporeal membrane oxygenation (ECMO)
- Fever: First aid
- Fever treatment: Quick guide to treating a fever
- Honey: An effective cough remedy?
- How do COVID-19 antibody tests differ from diagnostic tests?
- How to take your pulse
- How to measure your respiratory rate
- How to take your temperature
- How well do face masks protect against COVID-19?
- Is hydroxychloroquine a treatment for COVID-19?
- Loss of smell
- Mayo Clinic Minute: You're washing your hands all wrong
- Mayo Clinic Minute: How dirty are common surfaces?
- Multisystem inflammatory syndrome in children (MIS-C)
- Nausea and vomiting
- Pregnancy and COVID-19
- Safe outdoor activities during the COVID-19 pandemic
- Safety tips for attending school during COVID-19
- Sex and COVID-19
- Shortness of breath
- Thermometers: Understand the options
- Treating COVID-19 at home
- Unusual symptoms of coronavirus
- Vaccine guidance from Mayo Clinic
- Watery eyes
Related information
- COVID-19 vaccines: Get the facts
- How well do face masks protect against coronavirus?
- Post-COVID Recovery
News on coronavirus disease 2019 (COVID-19)
Learn the latest medical news about COVID-19 on Mayo Clinic News Network.
- Fight coronavirus COVID-19 transmission at home
Your gift holds great power – donate today!
Make your tax-deductible gift and be a part of the cutting-edge research and care that's changing medicine.
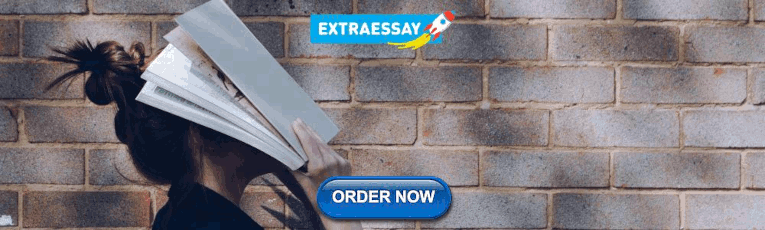
IMAGES
COMMENTS
Achieving a thorough understanding of the determinants of household water consumption is crucial to support demand management strategies. Yet, existing research on household water consumption ...
Plain water consumption varies by age, race/ethnicity, and socioeconomic status. During 2015-2018, US children and adolescents drank an average of 23 ounces of plain water daily, and US adults drank an average of 44 ounces. Among US children and adolescents, plain water intake is significantly lower in younger children, non-Hispanic Black ...
2. Representative Research Evidence. As shown in Table 2, a variety of methods and theoretical approaches have influenced our present understanding and theories regarding human water intake, euhydration, hypohydration, and water requirements.The range of measured or calculated variables includes dietary macronutrients, 24-h TWI (defined above), biomarkers of hydration status, water volumes (i ...
The subjects drank one bottle of water (550 mL) within 2 h of waking, and one bottle (550 mL) 2 h before bedtime. Subjects increased mean fluid intake from 1.3 L/day to 2.0 L/day. The major effects of water supplementation in this study were a decrease in blood pressure and an increase in body temperature.
The Food and Agriculture Organization of the United Nations categorizes water stress in the following ways: if withdrawals are less than 25 percent of resources, then a country has no water stress; 25-50 percent is low stress; 50-75 percent is medium; 75-100 percent high stress; and greater than 100 percent is critical stress. 3.
1 Introduction. Water conservation by households commonly involves behaviors to reduce water consumption. These behaviors are generally enacted through curtailment or efficiency activities (Abrahamse & Steg, 2009; Fielding et al., 2012).Curtailment includes fixing leaking taps, reusing grey water, taking showers rather than baths, turning off taps while brushing teeth, and using dishwashers ...
The predictive explanatory power of SEM for reducing water use increased from 44% to 50% after adding drought risk perception variable. In terms of quantification of elastic water use behavior, the average total water consumption in summer and winter were 71.3L/ (p.d) and 52.9L/ (p.d) under drought conditions, while it were 124.3 L/ (p.d) and ...
Resource management, particularly water, is a key challenge to urban sustainability. The supply of adequate water is essential to ensure healthy urban living, but access to clean water still evades millions of people around the globe (WHO & UNICEF 2021).At a basic level, water is required for direct consumption, food preparation as well as for hygiene purposes.
While there is an abundance of research on water consumption and conservation drivers and determinants in the literature (Basani and Reilly, 2008; House-Peters and Chang, 2011; Matos et al., 2014; Lowe et al., 2015; Makki et al., 2015), studies on the implicit attitudes that drive consumers' water consumption behaviours are very scanty. 5.1.
The research hypothesis is that water consumption records in their original or transformed form can provide a basis for aggregating the data set into groups of consumers with similar consumption characteristics. This paper presents a methodology for water consumption data clustering by comparing several data representation methods (termed ...
This literature review was based on research related to water consumption in different regions. To understand this water demand, we first analysed the urban water availability and the global water situation. Then, a second analysis was developed to verify the factors that influence such consumption in different building types and contexts, such ...
The ratio of biomass accumulation per unit water consumption is known today as water use efficiency (WUE) and is widely relevant to agriculture (e.g. Blum 2009; Tallec et al. 2013; Vadez et al. 2014), to forest ecology (e.g. Linares and Camarero 2012; Lévesque et al. 2014) and in the context of global climate change (Cernusak et al. 2019).This ratio can be defined at various levels, from the ...
Perceived knowledge on personal water usage is positively related to the intention to save water.Past research focusing on water meters have proved that smart-water meters may reduce the household water consumption on an average rate of 19.6% (Sønderlund et al., 2014) while Davies et al. (2014) found out a reduction of 1.05 kl per month in ...
Water consumption data for Europe are collected far more selectively than even the crude water intake questions from NHANES. The recent EFSA report provides measures of water consumption from a range of studies in Europe. 4, 105, 108, 109 Essentially what these studies show is that total water intake is lower across Europe than the United ...
Water resources in the Lima metropolitan area. Peru contains approximately 5% of the world's freshwater, with about 74,000 m 3 per person per year (Comité Nacional del Libro Azul para el Perú, Citation 2016, p. 36). Footnote 2 In 2008, of the total amount of extracted water, 88.7% was used for agricultural purposes, 9.2% for municipal use, and 2.1% for industrial use (including 1.1% for ...
Water Resources Research is an AGU hydrology journal publishing original research articles and commentaries on hydrology, water resources, and the social sciences of water. Abstract Under current global warming and accelerated population growth scenarios, cropland irrigation water consumption has become a central issue limiting the ...
Water Research publishes refereed, original research papers on all aspects of the science and technology of the anthropogenic water cycle, water quality, and its management worldwide. A broad outline of the journal's scope includes: •Treatment processes for water and wastewaters (municipal, agricultural, industrial, and on-site treatment ...
Research efforts aimed at quantitatively comparing water demand data (water consumption volumes, peaks, patterns) gathered across different scales and geographical contexts would advance the generalization of water demand models and contribute to upscale the findings from currently localized water demand studies.
This coefficient is based on the efficient water consumption per use (in l/use) provided by the user and on the standard water consumption per use (in l/use) derived from the small-scale survey. For example, if water-efficient washing machines consume 42 l per use, the corresponding efficiency coefficient is 42/57.7 ≈ 0.73 , since washing ...
New research, published March 28, 2024, shows how Colorado water is used. (Brian Richter, Contributed) A new, improved water budget. Richter worked with 11 other researchers from universities around the country to compile what they say is the first complete accounting of water use in the Colorado River Basin.
By uncovering the intricate influences of climate, land use, and CO 2 on terrestrial water storage over long-time period, this research enriches our comprehension of global hydrological shifts, informs strategies for water resource resilience, and provides valuable insights for environmental stakeholders and researchers.
Syracuse, N.Y. - April 2, 2024 - Until now, groundwater - a critical water resource around the globe, especially in dry regions - has been largely unstudied in its importance and role in sustaining ecosystems.A new groundbreaking research effort led by the SUNY College of Environmental Science and Forestry (ESF) in partnership with University of California Santa Barbara (UCSB), Cardiff ...
Saudi Arabia (SA) is one of the world's arid, most water-scarce nations without permanent water resources. The purpose of this article is to provide a comprehensive overview of Saudi Arabia's water resources availability and reliability in terms of water supply, demand, and the major challenges that water faces. Saudi has an annual water supply of roughly 89.5 m3 per person as consumption ...
04/05/2024. Dr. Charles Werth is a new ARPA-E Program Director. His research prior to joining ARPA-E focused on the intersection of mass transport, fluid flow, interfacial and geo-chemistry, redox reactions, microbial physiology, and material science for the development of sustainable water treatment, groundwater remediation, and geological ...
The use of water in the electricity system can be assessed using multiple metrics. The most common measure is the volumetric water footprint. It includes direct (i.e. water use for cooling at the point of generation) and indirect water use (i.e. upstream water use in the supply chain of fuels or equipment).
New research could help stakeholders bring water use along the Colorado River back in sync with what nature provides. In Wyoming and other Upper Basin states, 90% of irrigated farming is used to feed cattle, which accounts for more than three times the water use of all cities, towns, commercial and industrial uses combined.
The virus spreads when other people breathe in infected droplets or when the droplets land in their eyes, noses or mouths. The COVID-19 virus also can spread if people touch their eyes, noses or mouths after touching a surface with the virus on it. Without cleaning and disinfection, the COVID-19 virus may stay on surfaces from hours to days.