
An official website of the United States government
The .gov means it’s official. Federal government websites often end in .gov or .mil. Before sharing sensitive information, make sure you’re on a federal government site.
The site is secure. The https:// ensures that you are connecting to the official website and that any information you provide is encrypted and transmitted securely.
- Publications
- Account settings
Preview improvements coming to the PMC website in October 2024. Learn More or Try it out now .
- Advanced Search
- Journal List
- Int J Mol Sci

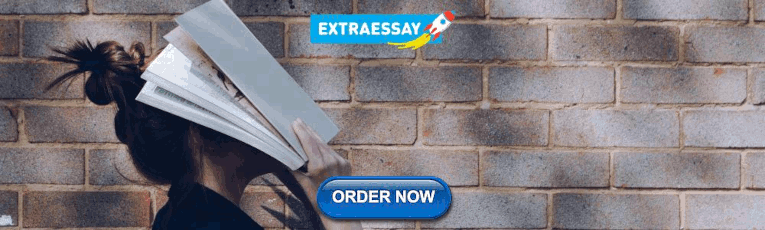
Polycystic Ovary Syndrome: A Comprehensive Review of Pathogenesis, Management, and Drug Repurposing
Hosna mohammad sadeghi.
1 Toxicology and Diseases Group (TDG), Pharmaceutical Sciences Research Center (PSRC), The Institute of Pharmaceutical Sciences (TIPS), Tehran University of Medical Sciences, Tehran 11369, Iran; moc.liamg@9731smansoh (H.M.S.); moc.oohay@adya_ileda (I.A.); ri.ca.smut.tneduts@ivasuoM-ts (T.M.); moc.liamg@57ilainadheizram (M.D.)
2 Department of Toxicology and Pharmacology, Faculty of Pharmacy, Tehran University of Medical Sciences, Tehran 11369, Iran
Daniela Calina
3 Department of Clinical Pharmacy, University of Medicine and Pharmacy of Craiova, 200349 Craiova, Romania
Anca Oana Docea
4 Department of Toxicology, Faculty of Pharmacy, University of Medicine and Pharmacy, Petru Rares, 200349 Craiova, Romania; moc.liamg@00anaoad
Taraneh Mousavi
Marzieh daniali, shekoufeh nikfar.
5 Department of Pharmacoeconomics and Pharmaceutical Administration, Faculty of Pharmacy, Tehran University of Medical Sciences, Tehran 11369, Iran; [email protected]
6 Personalized Medicine Research Center, Endocrinology and Metabolism Research Institute, Tehran University of Medical Sciences, Tehran 11369, Iran
7 Evidence-Based Evaluation of Cost-Effectiveness and Clinical Outcomes Group, Pharmaceutical Sciences Research Center (PSRC), The Institute of Pharmaceutical Sciences (TIPS), Tehran University of Medical Sciences, Tehran 11369, Iran
Aristidis Tsatsakis
8 Department of Analytical and Forensic Medical Toxicology, Sechenov University, 119991 Moscow, Russia; moc.liamg@sikastastsira
9 Department of Forensic Sciences and Toxicology, Faculty of Medicine, University of Crete, 71003 Heraklion, Greece
10 Laboratory of Toxicology, Medical School, University of Crete, 70013 Heraklion, Greece
Mohammad Abdollahi
Polycystic ovary syndrome (PCOS) is an endocrine-gynecology disorder affecting many women of childbearing age. Although a part of the involved mechanism in PCOS occurrence is discovered, the exact etiology and pathophysiology are not comprehensively understood yet. We searched PubMed for PCOS pathogenesis and management in this article and ClinicalTrials.gov for information on repurposed medications. All responsible factors behind PCOS were thoroughly evaluated. Furthermore, the complete information on PCOS commonly prescribed and repurposed medications is summarized through tables. Epigenetics, environmental toxicants, stress, diet as external factors, insulin resistance, hyperandrogenism, inflammation, oxidative stress, and obesity as internal factors were investigated. Lifestyle modifications and complementary and alternative medicines are preferred first-line therapy in many cases. Medications, including 3-hydroxy-3-methyl-3-glutaryl-coenzyme A (HMG-CoA) reductase inhibitors, thiazolidinediones, sodium-glucose cotransporter-2 inhibitors, dipeptidyl peptidase-4 inhibitors, glucose-like peptide-1 receptor agonists, mucolytic agents, and some supplements have supporting data for being repurposed in PCOS. Since there are few completed clinical trials with a low population and mostly without results on PCOS repurposed medications, it would be helpful to do further research and run well-designed clinical trials on this subject. Moreover, understanding more about PCOS would be beneficial to find new medications implying the effect via the novel discovered routes.
1. Introduction
Polycystic ovary syndrome (PCOS) is a heterogeneous endocrine disorder that impacts many women of the reproductive age worldwide [ 1 ]. This syndrome is often associated with enlarged and dysfunctional ovaries, excess androgen levels, resistance to insulin, etc. [ 2 ]. It is estimated that approximately every 1 in 10 women face PCOS before menopause and struggle with its complications [ 3 ].
Although the high ratio of luteinizing hormone (LH) to follicle-stimulating hormone (FSH) and increased frequency of gonadotropin-releasing hormone (GnRH) is known as the underlying causes of PCOS [ 4 ], the exact etiology and pathology have not been comprehensively well-known [ 4 , 5 ]. Evidence suggests the role of different external and internal factors, including insulin resistance (IR), hyperandrogenism (HA), environmental factors, genetic, and epigenetics. In addition, it is worth mentioning that PCOS increases the risk of further complications like cardiovascular diseases [ 5 , 6 ], type 2 diabetes mellitus [ 5 , 6 ], metabolic syndrome [ 6 ], depression, and anxiety [ 7 ].
To manage this condition, the most crucial step is to lose at least 5% of the weight; therefore, having a regular exercise plan and fat and sugar-free diets are also recommended to every woman with PCOS. Furthermore, in some cases, taking complementary and alternative medicine strategies with or without other treatments is preferable due to their prior beliefs, lower costs, etc.
Physicians tend to use (combined) oral contraceptives, antiandrogen agents, insulin sensitizers, and ovulation inducers [ 4 ]. Up until today, there is no United States Food and Drug Administration (USFDA) approved medication specifically for PCOS, and all mentioned medications are used off-label [ 8 ]. Apart from the essential need for improvement in the research and development of new drug molecules and new drug discovery, novel medications could be found with drug repurposing methods [ 9 ]. On this very spot, there are plenty of medications, previously approved by USFDA for indications rather than PCOS; and, today, there is a desire to implement them as the therapeutic options in the management of PCOS.
These agents vary from anti-diabetic medications such as pioglitazone, empagliflozin, sitagliptin, liraglutide to 3-hydroxy-3-methyl-3-glutaryl-coenzyme A (HMG-CoA) reductase inhibitors like simvastatin and atorvastatin, as well as mucolytic drugs like N-acetyl cysteine.
Given that PCOS is a growing issue that is unfortunately followed by many unwanted complications and that available methods and medications are not 100% effective, it is essential to investigate its pathogenesis and find out new pharmacological targets carefully. This could be done through repositioning approaches, saving time and cost.
This review discusses PCOS’s definition, diagnosis, and etiology, focusing on the pathogenesis and management of this syndrome. Internal and external factors contributing to PCOS have been comprehensively studied, and several commonly prescribed medications with their complete drug information are provided. Subsequently, a couple of repurposed medications are mentioned thoroughly, reviewing the related clinical trials over the past five years.
PubMed, Google Scholar, ScienceDirect, TRIP database, and UpToDate were comprehensively searched for publications including PCOS relevant keywords in different areas, focusing on the new ones (since 2016) and excluding those with a language rather than English or animal studies. In addition, Clinicaltrials.gov was searched to find data about completed or running trials of repurposed drugs in PCOS over the past five years.
3. Diagnosis
PCOS is among the conditions that cannot be diagnosed with basic diagnostic tests, including blood tests, culture, and biopsy; thus, there is no certain test for PCOS diagnosis. Differential diagnosis is called excluding the relevant disorders according to the symptoms and narrowing the choices. In order to establish a differential diagnosis for PCOS, hyperprolactinemia, thyroid disease, Cushing’s syndrome, and hyperplasia of adrenal should be excluded based on the associated investigations [ 10 , 11 ]. Although considering past medical history, weight changes, and symptoms of insulin resistance might be helpful, pelvic examination, a transvaginal ultrasound, and measuring the level of hormones are among the most frequently recommended investigations [ 12 ]. According to the National Health Service (NHS), irregular or infrequent periods, high levels of androgenic hormones or symptoms, and scans showing polycystic ovaries are the specified criteria for PCOS [ 13 ]. In addition, Rotterdam PCOS diagnostic criteria in adults are the most commonly used method. In an ultrasound, the presence of two clinical or biochemical hyperandrogenism, ovulatory dysfunction, or polycystic ovaries would finalize a PCOS diagnosis [ 14 ].
4. Etiology and Risk Factors
4.1. external factors, 4.1.1. epigenetic mechanism.
Epigenetic refers to inheritable alterations in genome and gene expression without any changes in DNA sequence [ 15 , 16 ]. These changes involve adding or omitting chemical components on DNA or histone [ 17 ]. Increased LH activity is a seen phenomenon in PCOS women. It may relate to the problems in follicle development and HA, which are common among PCOS patients [ 18 ]. LH/choriogonadotropin receptor (LHCGR) is responsible for the steroidogenesis process in theca cells [ 19 ]. This receptor hypomethylation leads to higher gene expression and sensitivity to LH [ 18 , 20 ].
A study on PCOS patients approved that hypomethylated sites are related to overexpression of LHCGR [ 15 , 19 ] on theca cells surface [ 19 ]. In addition, epoxide hydrolase 1 (EPHX1) is an active enzyme in degrading aromatic compounds [ 15 , 19 , 21 ]. Its gene promoter hypomethylation [ 15 , 19 ] increases enzyme expression [ 15 ]. Overproduction of EPHX1 reduces the transformation of testosterone to estradiol, which can contribute to PCOS [ 15 ]. Furthermore, peroxisome proliferator-activated receptor gamma (PPAR-γ) plays a role in ovaries’ function [ 15 , 18 , 19 , 22 ]. Hypermethylation of PPARγ, hypomethylation of nuclear co-repressor 1 [ 19 , 22 ], and alteration in acetylation of histone deacetylase 3, for which both are PPARγ co-repressors [ 15 ], are observed in PCOS patients showing HA [ 15 , 19 , 22 ]. These alterations were noticed in PCOS women’s granulosa cells [ 18 , 23 ].
4.1.2. Environmental Toxicants
The United States Environmental Protection Agency (USEPA) defines endocrine-disrupting chemical (EDC) as “an exogenous agent that interferes with the synthesis, secretion, transport, binding, action, or elimination of natural hormones in the body that are responsible for the maintenance of homeostasis, reproduction, development and/or behavior” [ 24 ].
EDCs may act as hormones’ agonists or antagonists in binding to their receptors [ 25 ]. EDCs are almost parts of everything we use in our daily life [ 21 ]. Their structures consist of phenols or halogens like chlorine and bromine, so they imitate steroid hormones’ actions [ 21 ]. Studies have approved the higher serum concentration of EDCs in PCOS suffering women [ 21 , 26 ]. Prolonged and continuous exposure to EDCs from prenatal to adolescence can cause susceptibility to PCOS [ 21 , 27 ].
As an example, bisphenol A (BPA) BPA is a synthetic compound used in polycarbonate plastics, epoxy resins [ 25 , 28 ], dental filling, food and drink packages [ 25 ], baby bottles, and polyvinyl chloride (PVC) [ 28 ], which affects metabolism through different pathways. BPA directly affects oogenesis [ 29 ] by interacting with estrogen receptor (ER) α and β, non-classical membrane ER, and G-protein coupled receptor 30 (GPCR30) [ 21 , 28 , 29 ]. It also triggers androgen secretion and restrains testosterone catabolism in theca cells [ 21 , 29 ].
Another effect of BPA on interstitial theca cells is the overproduction of androgens by dysregulation of 17β-hydroxylase (P450c17) [ 28 , 30 ], cholesterol side-chain cleavage enzyme (P450scc), and steroidogenic acute regulatory protein [ 30 ]. BPA’s influence on granulosa cells refers to reducing the expression of aromatase enzyme and production of estrogen [ 21 , 29 ]. Lastly, it disturbs the intrafollicular environment and damages the oocyte development and maturation [ 21 , 29 ]. BPA’s indirect effect on HA involves downregulation of testosterone 2a-hydroxylase and testosterone 6b-hydroxylase enzymes in liver level, and thus a higher concentration of testosterone [ 30 , 31 ].
In addition, BPA is a potent ligand for sex hormone-binding globulin (SHBG) and replaces testosterone; thereby, free testosterone concentration increases. Androgen and BPA have a two-way relation; high androgen inactivates the uridine diphosphate-glucuronosyl transferase enzyme and reduces BPA clearance in the liver. This process causes a high concentration of free BPA in blood and worsens its negative effects on the ovaries [ 21 , 29 , 30 , 31 ].
Additionally, it is believed that BPA may act as an obesogen [ 28 , 30 ]. Its obesogenic influence includes upregulation of adipogenesis-related genes [ 30 ], stimulation of adipocytes differentiation [ 28 , 30 ], potentiation of the accumulation of lipid in cells incorporated in medical syndrome, and triggering the conversion of target cells to adipocytes via phosphatidylinositol 3-kinase pathway [ 30 ].
Adipogenesis due to BPA happens because of the activation of the glucocorticoid receptor. Activation of the receptor upregulates the enzyme involved in the conversion of cortisone to cortisol, thus inducing adipogenesis [ 28 ]. Moreover, BPA prompts the release of interleukin-6 (IL-6) and tumor necrosis factor α (TNF-α) [ 30 , 31 ], both involving adiposity and IR [ 30 ]. In addition, it restrains the release of adiponectin [ 28 , 29 , 30 , 31 ] and the beneficial compound in protecting against IR [ 28 , 30 , 31 ].
It can also change glucose homeostasis [ 28 , 29 , 31 ] by directly influencing the pancreatic cells [ 29 ]. BPA causes a chronic increase in insulin and further IR in long exposure [ 30 ] by affecting the mitochondrial activity and metabolic pathways of β-pancreatic cells [ 28 ]. BPA reduces glucagon secretion by inhibiting the intracellular calcium ion fluctuating pattern with a lack of glucose condition [ 30 ].
Advanced glycation end products (AGEs), also called glycotoxins, are another chemical group affecting body health. AGEs are pro-inflammatory molecules [ 21 , 23 , 29 , 32 ] that interact with their surface receptor called RAGE (receptor for AGE) [ 21 , 23 , 29 ] and stimulate pro-inflammatory pathways and oxidative stress [ 21 , 23 , 29 , 32 ]. AGEs can be absorbed into the body as exogenous compounds or derived from nonenzymatic glycation and oxidation of proteins and lipids [ 21 ]. Increased concentration of AGEs in serum has been detected in PCOS patients [ 21 ]. AGEs interrupt pre-ovulatory follicles growth via ERK1/MAPK pathway and damage follicles by oxidative stress caused by interaction with RAGEs [ 21 ]. This interaction increases intracellular inflammatory molecules [ 21 ].
In vitro studies on 3T3-L1 cell lines showed that glycotoxins are likely to trigger adipogenesis [ 21 ]. On the other hand, a higher body mass index corresponds to a lower extent of soluble RAGEs, which is responsible for glycotoxin clearance and deposition of AGEs in the reproductive system, especially in ovaries [ 21 , 29 ]. This bilateral relation worsens inflammatory processes and metabolic syndrome in PCOS [ 21 ]. AGEs also play a role in IR [ 21 , 29 ]. These compounds disrupted glucose transport in the human granulosa KGN cell line [ 21 ] and reduced glucose uptake by adipocytes in previous research [ 21 , 29 ]. They also involve IR by causing oxidative stress, inflammation, and glycation of proteins, which considerably diminishes insulin sensitivity [ 21 ]. Moreover, increased concentration of AGEs changes the insulin signaling pathway and interferes with glucose transporter 4 (GLUT-4) translocation [ 23 ].
4.1.3. Physical and Emotional Stress
Although there is minimal information on the role of stress in PCOS, it is known that PCOS possesses adverse effects on self-esteem and mental health. Chronic stress results in hypertrophy and hyperplasia of adipocytes. This phenomenon happens as a result of glucocorticoids’ effect on pre-adipocytes maturation. Chronic stress is also associated with adipokine secretion, attraction, and activation of stromal fat immune cells [ 33 ].
In addition, it is responsible for making an inflammatory condition by leading to high levels of inflammatory cytokines like IL-6 and TNF-α, along with disrupting oxidant-antioxidant balance [ 33 ]. In addition, chronic stress plays a vital role in IR.
Stress triggers the hypothalamic-pituitary-adrenal (HPA) axis to release cortisol [ 34 , 35 ]. Cortisol leads to IR by stimulating visceral fat accumulation, gluconeogenesis, and lipolysis [ 35 ]. Moreover, cortisol arouses glucose production in the liver [ 35 ]. Stress is also involved in enhancing insulin levels [ 34 ]. Other stress influences on PCOS may refer to inference with anti-mullerian hormone (AMH) and changing sex hormone levels [ 34 , 35 ].
4.1.4. Diet
Although nutrition contributions to PCOS is unclear, studies showed a relationship between some nutrient levels and PCOS indices.
Saturated fatty acids (SFAs) intake plays a role in PCOS by producing an inflammatory status [ 36 ] and reducing insulin sensitivity [ 37 ]. Taking SFAs induces inflammation by triggering an increase in TNF-α level in circulation and expressing a specific cytokine suppressor [ 36 ].
Vitamin D deficiency may exacerbate PCOS [ 37 , 38 ] or the comorbidities induced by PCOS [ 38 ]. Calcitriol upregulates insulin receptors at mRNA and protein levels. It also increases insulin sensitivity directly and indirectly. The direct effect occurs by activating PPAR-δ, the involved receptor in fatty acids metabolism in adipose tissue and skeletal muscle. The indirect impact is the regulation of intracellular calcium, which is vital for insulin-mediated signaling in fat and muscle [ 38 ]. On the other hand, vitamin D deficiency may result in insulin resistance by causing an inflammatory response [ 37 , 39 ]. Furthermore, vitamin D downregulates the AMH promoter [ 39 ].
4.2. Internal Factors
4.2.1. insulin resistance.
IR means an insufficient cells response to insulin [ 40 ]. IR is independent of patients’ adiposity, body fat topography, and androgen levels [ 18 , 41 ]; i.e., it has been reported in lean patients as well [ 18 , 42 ]. It should be mentioned that IR is tissue-selective in PCOS women [ 18 , 43 ], although skeletal muscles [ 18 , 43 , 44 ], adipose tissue, and liver lose their sensitivity to insulin, adrenal glands [ 18 , 43 ], and ovaries remain sensitive [ 18 , 28 , 43 , 45 ].
Insulin directly triggers androgens production in ovarian theca cells [ 32 , 44 , 46 , 47 , 48 ] and grow [ 48 ]. Insulin effectively stimulates ovarian follicle growth and hormone secretion by stimulating its receptors in the follicle membrane cells [ 49 ]. It also triggers ovarian P450c17 [ 18 , 23 , 50 ] and P450scc enzyme activity to promote ovarian steroidogenesis [ 18 , 51 ] and increases them with the synergistic effect of chorionic gonadotropin [ 52 ]. This hormone, as well as insulin-like growth factor 1 (IGF-1) [ 18 ], synergizes with luteinizing hormone [ 18 , 45 ]. Hyperinsulinemia increases LH-binding sites and androgen-producing response to LH [ 44 ]. LH and insulin interaction enhance steroidogenic acute regulatory enzyme and CYP450c17 mRNA expression [ 52 , 53 ]. CYP450c17 is involved in androgen production [ 23 , 44 ]. Likewise, IR independently enhances CYP17A1 activity, the productive enzyme in androstenedione and testosterone production [ 52 ].
On the other hand, hyperinsulinemia reduces hepatic SHBG [ 18 , 32 , 40 , 49 , 52 , 54 , 55 , 56 ], increasing free testosterone levels in blood [ 18 , 32 , 52 , 54 , 56 ]. In addition, hyperinsulinemia inhibits IGF-1 binding protein production in the liver. IGF-1 is responsible for triggering the production of androgens in thecal cells. Inhibition of the production of IGF-1 binding proteins leads to a higher concentration of this substance in blood circulation and then higher production of androgens in thecal cells [ 18 , 46 ]. Moreover, IGF-1 upregulation decreases a specific miRNA and thus accelerates granulosa cells apoptosis and inhibits folliculogenesis [ 52 ]. HA [ 46 ] and hyperinsulinemia [ 45 , 46 , 57 ] both play a role in stopping follicles growth [ 45 , 46 ]. This stoppage is attributed to menstrual irregularity, anovulatory sub-fertility, and amassing of immature follicles [ 46 ].
Furthermore, hyperinsulinemia contributes to PCOS by affecting the pituitary gland. Excessive insulin stimulates its receptors in the pituitary gland to release LH [ 49 ]. Accumulation of insulin stimulates GnRH and LH pulse secretion via influencing both amplitude and frequency [ 23 ]. Insulin’s indirect effect on PCOS is augmented by pituitary gonadotropin sensitivity to GnRH [ 18 ], and hyperinsulinemia increases GnRH neuron activity [ 58 ].
The insulin’s influence on adipose tissue and inflammation is another essential PCOS pathogenesis topic. Insulin stimulates adipogenesis and lipogenesis and inhibits lipolysis [ 42 ], resulting in fat accumulation [ 44 ]. IR leads to enhanced plasma levels of free fatty acids (FFAs), affecting the liver and adipose tissue [ 32 ]. Moreover, IR causes a reduction in omentin level independent of the patient’s body mass index (BMI). In addition, hyperglycemia can lead to inflammation by producing TNF-α from mononuclear cells (MNCs) [ 50 ].
4.2.2. Hyperandrogenism
Generally, hyperandrogenism (HA) reduces the SHBG level, leading to a higher concentration of free testosterone [ 18 , 59 ]. It was observed that PCOS women have higher concentrations of testosterone in plasma which can convert to estrone in adipose tissue. Increased alteration of estrone to estradiol affect follicle growth and increases the LH to FSH ratio causing ovulatory dysfunction [ 23 ].
HA can result in AMH upregulation, which inhibits ovulation and the development of follicles by a different mechanism. Furthermore, the IGF-II level is negatively related to androgen levels, and HA reduces IGF-II in follicular fluid. IGF-II positively relates to follicle diameters and estradiol concentration in follicular fluid [ 23 ]. In addition, HA increases LH indirectly [ 58 , 60 ]. Estradiol and progesterone are responsible for GnRH and LH secretion via negative feedback [ 58 , 61 , 62 ]. HA disrupts the negative feedback on secretion [ 18 , 23 , 61 , 62 ] resulting in increased LH levels [ 18 , 62 ]. Interaction of androgen and its receptor interferes with progesterone receptor transcription. Moreover, this receptor is involved in converting high levels of androgens to compounds that modulate the gamma-aminobutyric acid A (GABA A ). Modulation of the GABA A receptor triggers GnRH neurons and weakens the response to negative progesterone feedback [ 58 ]. In addition, it is assumed that androgens might decrease hepatic nuclear factor-4α (HNF-4α) levels by inhibiting lipid synthesis. HNF-4α stimulates SHBG expression by binding to its promoter [ 63 ].
HA contributes to other influential factors of PCOS, including IR, inflammation, and oxidative stress.
HA aggravates IR via different routes; it reduces the insulin sensitivity, expression of GLUT-4 and inhibits insulin degradation in the liver [ 23 , 32 ]. Moreover, HA increases a type of skeletal muscle fibers that have low insulin sensitivity [ 32 ]. On the other hand, HA worsens central adiposity, which is involved in IR [ 23 , 32 ]. Additionally, it was observed that testosterone increases inflammatory chemicals such as lipopolysaccharide-induced IL-6 in 3T3-L1 adipocytes by activating some signaling pathways [ 64 ]. One way androgen results in oxidative stress is by increasing MNC sensitivity to glucose and aggravating glucose-stimulated oxidative stress [ 65 ]. It is worth mentioning that dehydroepiandrosterone as an androgen decreases interferon-γ (IFN-γ), an essential regulator in normal ovarian physiology and cell function [ 64 ].
In addition, it should be mentioned that studies on PCOS women approved the resemblance of their fatty tissue to men, and hence the effect of HA on adipose tissue dysfunction [ 8 ]. In addition, HA is a cause of adipocyte hypertrophy and consequential damages to adipokine secretion [ 55 ].
4.2.3. Inflammation
Appropriate inflammation is a vital cause of oocyte growth and ovulation [ 66 ]. However, high levels of white blood cell [ 46 , 66 ], C-reactive protein (CRP) [ 4 , 46 , 50 , 66 , 67 ], and other inflammatory biomarkers in peripheral blood are associated with PCOS [ 4 , 46 , 66 , 67 , 68 ]. Inflammation is a cause of HA [ 44 , 69 ]. TNF-α is a pro-inflammatory chemical that can worsen IR. Contribution to IR happens due to interference of pro-inflammatory molecules with insulin signaling pathways [ 32 , 67 ] and reduction of GLUT-4 expression [ 23 ]. Some studies showed that the insulin receptor substrate (IRS) serine residue phosphorylation inhibits insulin receptor signaling [ 32 , 70 ]. This phenomenon results in the prevention of GLUT-4 translocation and glucose reuptake [ 70 ]. In addition, TNF-α showed the ability to prompt theca cells proliferation in vitro [ 71 ]. Furthermore, IL-1 hinders the FSH and LH receptors. Inhibition of these receptors leads to inhibition of follicular development and ovulation [ 66 ]. Both TNF-α and IL-1β inhibit activation of HNF-4α by different mechanisms [ 23 ]. In addition, NLRP3 inflammasomes induce follicular pyroptosis, ovarian fibrosis, and disturbance of follicular formation [ 66 ]. An increase in CRP level is another cause of IR in insulin-sensitive tissues. IR occurs because of increased pro-inflammatory factors secreted by the liver and monocytes. CRP stimulates this increase in secretion [ 72 ]. Moreover, another study approved the higher-than-normal level of IL-6 mRNA in granulosa cells [ 66 ].
4.2.4. Oxidative Stress
Oxidative stress (OS) is an imbalance between pro-oxidants and antioxidants [ 71 , 72 , 73 ]. Oxidative molecules include different chemicals such as reactive oxygen species (ROS) [ 73 , 74 , 75 ] (e.g., O 2− , H 2 O 2 , and OH − ) [ 76 ] and reactive nitrogen species (RNS) [ 74 , 75 ]. ROS plays a role in different mechanisms like signaling pathways [ 71 , 73 , 76 ], cell growth [ 71 , 73 ], and differentiation, as well as RNS [ 73 ]. RONS also acts on ovaries functions like steroidogenesis [ 67 , 77 ] and affects neurons responsible for feeding behavior to induce hunger [ 71 ]. Overproductions of oxidative chemicals cause various damage to vital molecules such as lipids, proteins, and DNA [ 73 , 74 , 75 , 77 ].
Increased OS has been seen in PCOS patients in different studies [ 74 , 78 , 79 ]. Increased levels of OS activate the nuclear factor-kappa B (NF-κB) [ 72 , 75 ]. NF-κB is involved in inflammatory pathways [ 75 ] and affects the production of pro-inflammatory cytokines like TNF-α and IL-6 [ 72 , 80 ]; the effect in IR and PCOS was explained above. A high level of OS also increases the release of TNF-α [ 77 ]. On the other hand, increased OS actuates some protein kinases that trigger serine/threonine phosphorylation instead of normal tyrosine phosphorylation of IRS. Thus, the insulin signaling pathway is inhibited, and OS leads to IR [ 67 ]. OS also plays a role in obesity. It increases mature adipocyte size and consequently stimulates pre-adipocyte proliferation and adipocyte differentiation. OS also imposes a major effect on obesity [ 71 ].
4.2.5. Obesity
Obesity is a key in low-grade chronic inflammation [ 72 ]. Accumulation of adipocytes in visceral fat leads to hypoxia and consequent necrosis, which causes inflammatory cytokines production [ 66 ]. Adipocyte death due to hypertrophy causes an inflammatory state [ 44 , 69 ]. The mononuclear cells of adipose tissue produce pro-inflammatory cytokines [ 6 , 44 , 81 ]. Excess abdominal fat is also responsible for the inflammatory condition [ 6 , 44 , 81 ].
Obesity also plays a role in hyperinsulinemia, IR, and HA occurrence. Visceral obesity arouses an increase in non-esterified fatty acids (NEFAs) levels in the blood. Skeletal muscles uptake NEFAs as the energy source instead of glucose. This hyperglycemia leads to a pancreas rapid reaction and hyperinsulinemia [ 55 ]. In addition, the lipolytic response of visceral fat to catecholamines causes lipotoxicity [ 44 ] and impairment of insulin clearance and activity [ 81 ].
FFA stimulates IRS-1 serine/threonine phosphorylation and reduces tyrosine phosphorylation. Increased FFAs reduce insulin and glucose uptake sensitivity in intramyocellular lipids [ 52 ]. Notably, that visceral fat is weightier in IR than abdominal [ 44 ] and subcutaneous fat [ 81 ] as the visceral fat lipolytic response to catecholamines is more severe [ 44 , 81 ]. The reason is the increased function of the β3 and higher expression of β1 and β2 receptors [ 81 ]. Moreover, the type 1 isoenzyme of 11β-hydroxysteroid dehydrogenase (11β-HSD) is involved in converting cortisone to active cortisol, which is highly expressed in adipose tissue, especially in adipose tissue visceral ones. Glucocorticoids reduce glucose uptake and insulin signaling in omental adipocytes [ 81 ]. In addition, visceral fat’s adiponectin secretion is less than subcutaneous fats, and this phenomenon leads to decreased adiponectin secretion in obesity [ 46 ].
In addition to all adipose tissue’s functions mentioned above, this tissue has endocrine function and secretes chemicals called adipokines or adipocytokines. Adipocytes produce leptin, a high concentration of which inhibits the expression of aromatase mRNA in granulosa cells—thus interrupting androgens to estrogen conversion [ 52 ]. In addition, it is suggested that increased leptin levels are related to the absence of folliculogenesis [ 81 ]. Moreover, adiponectin, secreted by adipocytes [ 52 ], has insulin-sensitizing, anti-diabetic, and anti-inflammatory effects [ 46 ]. The adiponectin insulin-sensitizing effect causes a reduction in FFA uptake and gluconeogenesis. It also plays a role in progesterone and estrogen production, ovulation, and decreased GnRH secretion [ 52 ]. Furthermore, adiponectin reduces LH secretion from the pituitary, triggers estradiol secretion in granulosa, and is associated with androgen production in ovaries [ 81 ]. Omentin-1, another adipose tissue secreted chemical, improves IGF-1-induced progesterone and estradiol secretion in different ways, including increasing the steroidogenic acute regulatory protein and CYP450 aromatase expression and enhancing IGF-1 receptor signaling [ 82 ].
Adipose tissue also has several enzymes responsible for converting androstenedione to testosterone and testosterone to dihydrotestosterone [ 45 ]. 17β-HSD converts androstenedione to testosterone [ 44 , 81 ] and estrone to estradiol [ 81 ]. This enzyme is expressed in adipose tissue [ 44 , 81 ]. As a result of this process, excess adiposity exacerbates HA [ 45 ].
Furthermore, the accumulation of lipid in non-adipose tissues, called lipotoxicity, causes oxidative/endoplasmic reticulum stress linked with inflammation and IR. Excess fatty acids in muscles and liver induce IR via serine phosphorylation of insulin receptor by diacylglycerol [ 83 ]. In addition, lipid accumulation in the liver diminishes HNF-4α levels leading to reduced SHBG production [ 63 ].
A summary of the most representative molecular mechanisms of PCOS pathogenesis is presented in Figure 1 .

Summarized scheme regarding the pathophysiology of PCOS. Abbreviations and symbols: ↑ (increased), ↓ (decreased), DNA (deoxyribonucleic acid), GnRH (Gonadotropin-releasing hormone), IL-6 (interleukin 6), IR (insulin resistance), LH (luteinizing hormone), PCOS (polycystic ovary syndrome), SHBG (sex hormone-binding globulin), TNF-α (tumor necrosis alpha).
5. Management
The management approach and selection of the best therapy option depend on the target patient and her priorities [ 4 ]. The complications may vary from seeking fertility, regulation of menstrual disturbances to weight reduction or relief from hyperandrogenic symptoms, including acne, hirsutism, or androgenic alopecia [ 84 ]. Indeed, the approach should be individualized for each person to meet the optimal result [ 8 ]. There is no one ideal treatment for all women diagnosed with PCOS, which leaves physicians no choice but symptomatic therapy [ 85 ].
5.1. Lifestyle Modification and Non-Pharmacological Approaches
5.1.1. weight loss.
Elevated androgenic hormone levels lead to weight gain in women with PCOS, mainly in the abdominal area. As a result, many PCOS women have an apple shape body instead of a pear shape [ 37 ]. The first step for women diagnosed with PCOS would be weight reduction and calorie intake restriction [ 86 ]. Many studies demonstrate that even a 5% to 10% reduction in weight can restore the regular menstruation cycle [ 87 ]. For obese women, it would be best if they could reach their normal range of body mass index (BMI). Along with weight loss, the level of free testosterone decreases, and the incidence of metabolic syndrome reduces [ 84 ].
5.1.2. Diet
As mentioned above, to achieve specific goals for each woman, the best diet or nutrient regimen would be the tailored one [ 87 ]. However, some suggestions may help choose what to eat more or less. An ideal diet would be rich in fibers and low in saturated fats and carbohydrates. There is a carbohydrate classification considering the blood glucose response they cause within 2 h: low and high glycemic index carbohydrates. Low glycemic index carbohydrates are at the top of our agenda; they include foods and vegetables like broccoli, raw carrot, lentils, soy, bran breakfast cereals, whole-grain bread, etc. Patients should also be aware that foods with a high glycemic index for prevention, white rice, cakes and cookies, fries or chips, and some fruits such as pineapple or watermelon are actual examples [ 37 ].
5.1.3. Exercise
Exercise and physical activity play a key role in weight reduction. They may be beneficial to improve insulin sensitivity [ 88 ]. Different studies suggest various times for exercise during the week, but the American Heart Association (AHA) recommends approximately 150 min of moderate or 75 min of vigorous and intense exercise per week [ 84 ]. Several studies show that exercise, with or without being on a diet, can resume ovulation in women with PCOS. Exercise probably can affect ovulation through modulation of the hypothalamic-pituitary-gonadal (HPG) axis. In overweight and obese women, exercise leads to lower insulin and free androgen levels, inducing the restoration of HPA regulation of ovulation [ 88 ].
5.2. Complementary and Alternative Medicine (CAM)
Current management and accessible medications are only moderately effective in PCOS, and there are still some cases left untreated despite non-pharmacological and pharmacological treatments. Some literature claims that pharmacologically based therapies are only effective in 60% of patients [ 64 ]. Recent studies have demonstrated that using complementary and alternative medicine (CAM) as adjunctive therapy may benefit the management [ 89 ]. Today, CAM is a well-known approach that has been used at least at one point in more than 70% of PCOS patients during their diseases [ 90 ]. Several manners can categorize it; according to the latest edition of the National Center for Complementary and Integrative Health (NCCIH), complementary approaches can be classified by their primary therapeutic input into three classes of nutritional, psychological, physical, or all of them in combination [ 68 ]. One of the significant merits of CAM is that people often tend to accept these methods due to their beliefs and cultures; this leads to their improved adherence or tolerance to the therapy. Taking a look at prior studies, various methods of CAM including traditional Chinese medicine (TCM), immunotherapy, diet therapy (herbal and medicinal foods, probiotics, and vitamin or supplementation therapy), psychotherapy, spa, yoga, Tai Chi, and oxygen therapy have been considered as effective strategies in reducing the severity of PCOS and its complications [ 89 , 91 , 92 , 93 , 94 , 95 ]. Two critical subgroups of CAM effective in PCOS management are discussed in the following sections.
5.2.1. Acupuncture
Acupuncture, a fundamental part of CAM, has been used in China for more than 3000 years [ 89 ]. It is a kind of sensory stimulation in which thin needles are placed into the skin and muscles. Acupuncture improves clinical manifestations of PCOS by activating somatic afferent nerves of the skin and muscles, modulating somatic and autonomic nervous system activity and endocrine/metabolic functions [ 91 ]. Within acupuncture, β-endorphin production increases, affecting the secretion of gonadotropin-releasing hormone, ovulation, and menstrual cycle. This means that acupuncture may induce ovulation and restore the menstrual cycle [ 64 ].
5.2.2. Supplementations
Apart from medications with USFDA approval, plenty of supplementation products has been shown to be effective in some women with PCOS. These products include vitamin D supplements, resveratrol, α-lipoic acid, omega-3, berberine, folic acid, myoinositol (MI), and d-chiro-inositol (DCI).
Vitamin D is effective in several studies, especially in cold seasons of the year. The deficiency of this vitamin is thought to be important in the pathogenesis of PCOS, so just the compensatory amount would be suggested [ 96 ].
Resveratrol is among the most recommended supplements for the treatment of PCOS. It is assumed to possess chemopreventive, anti-inflammatory and antioxidant, cardioprotective, and neuroprotective effects [ 97 ]. Resveratrol may play a beneficial role in PCOS by inhibiting HMG-CoA reductase expression and activity, just like statins [ 98 ]. Clinical use of this product has been shown to reduce IR and the risk of type 2 diabetes development [ 99 ].
Alpha-lipoic acid and omega-3 are the two supplements found to improve women’s lipid profile and insulin sensitivity through their anti-inflammatory and antioxidant properties [ 4 ].
Berberine is a nutraceutical compound with possible, desirable effects against IR and obesity, particularly against visceral adipose tissue (VAT) [ 100 ]. Folic acid is usually an agent given to PCOS women seeking fertility [ 24 ].
Last but not least, MI and DCI are other essential and well-studied supplements for PCOS treatment. MI has been demonstrated to improve the activity of insulin receptors and can potentially restore the ovulatory function in most women with PCOS [ 85 ]. Inositol influences intracellular metabolic processes; it activates key enzymes controlling glucose’s oxidative and nonoxidative metabolism. Studies conducted on PCOS women taken MI alone, DCI alone, and these combinations of the two showed that they cause increased frequency of ovulation, decreased need for FSH therapy for triggering the ovulation, and a significant improvement in the pregnancy rate [ 97 ].
5.3. Pharmacological Treatments
Before heading to pharmacological approaches, healthy lifestyle advice must be given to all women diagnosed with PCOS regardless of their weight, complaint, or anything else. This is because, in most cases, and especially in mild to moderate forms, women can solely benefit from diet and exercise [ 101 ]. However, the treatment would rely mainly on the patient’s choices and condition in others. If the patient does not want to get pregnant and complains mostly about her menstruation irregularity, combined oral contraceptives (COCs) or progestins are the drugs of choice. The physician can choose the best oral contraceptive with a look on other symptoms rather than menstruation irregularity; for example, Yasmin ® , Yaz ® , or some other agents can show antiandrogenic effects and can, on the other hand, result in the reduction of androgen production. As a result, they might be helpful in those with hirsutism and/or acne complications.
Metformin, from the biguanides category, is usually prescribed along with the first-choice drugs (COCs) to restore the ovulation cycle in PCOS women because of its insulin sensitivity-increasing properties. Metformin has an antihyperandrogenic effect in the short term too.
In other patients who just want relief from dermatological manifestations due to hyperandrogenism, agents such as aldosterone receptor antagonists (e.g., spironolactone) and 5-alpha reductases (e.g., finasteride) would be more beneficial. Therapy options change for those with infertility who should take agents for ovulation induction like clomiphene citrate and/or aromatase inhibitors [ 84 ].
Of course, there are lots of limitations and precautions, and not everyone can benefit from the agents mentioned above owing to their adverse effects or contraindications. Many COC agents cause nausea and vomiting as they try to stimulate the pregnancy situation for the body. In addition, depression, headaches, and migraine are commonly seen in those taking them. Metformin also causes nausea and vomiting in the first days of consumption which may not be tolerated in all patients and leads to abandonment of the therapy. Spironolactone, a widely used and prescribed agent for androgen-related complications, can cause hyperkalemia. Therefore, it is suggested to look up the adverse reactions or contraindications in reliable drug literature or ask the patient’s history of any possible reaction before the prescription.
The complete list of the routine medications used to treat PCOS and the step-by-step treatment pathway considering the patient’s complaints are provided here in Table 1 .
Commonly prescribed medications in PCOS.
Abbreviations: COC: Combined oral contraceptives; DHT: Dihydrotestosterone; ER: Estrogen receptor; FSH: Follicle-stimulating hormone; GnRH: Gonadotropin-releasing hormone; HD: High dose; IM: Intramuscular; LD: Low dose; LH: Luteinizing hormone; PCOS: Polycystic ovary syndrome; PMS: Premenstrual syndrome; Ref: Reference; XR: Extended-release.
5.4. Drug Repurposing in PCOS
Drug repurposing, or in other terms drug repositioning or drug re-tasking, actually means finding new indications in other diseases or conditions for a medication that has previously been in the market and has USFDA approval for a specific therapeutic goal [ 9 ]. Using this method has shortened the duration of the research and development process, given the thought that the medicines have passed pre-clinical and clinical, safety, and immunological tests. As mentioned before in this review, PCOS still does not have a single ideal pharmacological treatment, and doctors typically tend to cure patients’ symptoms with other agents. Taking a look at other drugs—mostly diabetes agents—may be helpful to recognize some new medications for women with PCOS-related complications.
Table 2 and Table 3 , respectively, present general information and clinical trials of drugs primarily approved for other indications (e.g., diabetes type II, hyperlipidemia, weight reduction, etc.), which are now being examined to see their potential effect in PCOS.
Repurposed medications for the treatment of PCOS.
Abbreviations: CYP3A4: Cytochrome P450 3A4; DPP-4: Dipeptidyl peptidase 4; GI: Gastrointestinal; GLP-1: Glucose-like peptide 1; HIV: Human immunodeficiency virus; HMG-CoA: β-Hydroxy-β-methylglutaryl-CoA; MEN-2: Multiple endocrine neoplasia syndrome type 2; MTC: Medullary thyroid carcinoma; NYHA: New York Heart Association; Ref: Reference; SGLT-2: Sodium-glucose cotransporter-2; USFDA: United States Food and Drug Administration.
Clinical trials of the repurposed medications for PCOS since 2016.
Abbreviations: a.m.: before noon; AC: active comparator; AEs: adverse effects; AMH: anti-mullerian hormone; BID: twice a day; BMI: body mass index; CA: crossover assignment; DB: double-blind; Exp: experimental; FSH: follicle stimulation hormone; g: gram; IM: intramuscular; IU: international unit; LH: luteinizing hormone; mcg: microgram; mg: milligram; NA: not available; OL: open-label; P: placebo; PA: parallel assignment; PC: placebo-controlled; PCOS: polycystic ovary syndrome; PO: per oral (by mouth); QB: quadruple blind; QD: once a day; R: randomized; Ref: reference; SB: single-blind; SC: subcutaneous; SGA: single group assignment; TB: triple blind; TID: three times a day; Tx: treatment; UTI: urinary tract infection.
6. Conclusions
Although the pathogenesis of PCOS is not fully understood, it is believed that different factors from epigenetic alterations to obesity, inflammation, and inactivity may aggravate this syndrome. Since there is still no certain medication or definite cure for this condition, the routine approach after advising on some lifestyle modification and supplementary tips is symptomatic therapy with plenty of agents, including contraceptives, oral antidiabetics, or antiandrogens. In terms of the repurposing, there is a good chance that other approved agents could exert beneficial effects on PCOS. Since the complete profiles of these agents are available, and their efficacy and safety have already been comprehensively studied, the pathway for finding novel treatments becomes a little more straightforward. However, there is still very much to discover and examine for a better understanding of the pathogenesis, and, as a result, targeting the mechanism by proper medication.
Author Contributions
All authors made a significant contribution to the work reported, whether that is in the conception, study design, execution, acquisition of data, analysis, and interpretation, or in all these areas—that is, revising or critically reviewing the article; giving final approval of the version to be published; agreeing on the journal to which the article has been submitted; and confirming to be accountable for all aspects of the work. All authors have read and agreed to the published version of the manuscript.
This research received no external funding.
Conflicts of Interest
The authors have no relevant affiliations or financial involvement with any organization or entity with a financial interest in or financial conflict with the subject matter or materials discussed in the manuscript. This includes employment, consultancies, honoraria, stock ownership or options, expert testimony, grants or patents received or pending, or royalties.
Publisher’s Note: MDPI stays neutral with regard to jurisdictional claims in published maps and institutional affiliations.
Thank you for visiting nature.com. You are using a browser version with limited support for CSS. To obtain the best experience, we recommend you use a more up to date browser (or turn off compatibility mode in Internet Explorer). In the meantime, to ensure continued support, we are displaying the site without styles and JavaScript.
- View all journals
- Explore content
- About the journal
- Publish with us
- Sign up for alerts
- Journal Club
- Published: 20 February 2024
Neuroendocrinology
Beyond the ovary: rewiring our perspective on polycystic ovary syndrome
- Rebecca E. Campbell ORCID: orcid.org/0000-0002-0309-532X 1
Nature Reviews Endocrinology volume 20 , page 257 ( 2024 ) Cite this article
250 Accesses
18 Altmetric
Metrics details
- Endocrine reproductive disorders
- Neuroendocrine diseases
One in ten women of reproductive age is likely to have polycystic ovary syndrome (PCOS), a complex endocrine disorder that affects reproductive, metabolic, cardiovascular and psychological health. PCOS is defined by ovarian dysfunction, including the diagnostic features of androgen excess, reduced or absent ovulation, and polycystic ovarian morphology. However, two seminal studies in the late 1990s challenged the primary role of the ovary in PCOS, and instead implicated intrinsic features in the brain, specifically the gonadotropin-releasing hormone (GnRH) neuronal network, as central to PCOS pathology.
Usually, the reproductive axis features reciprocal communication between a network of neurons in the brain (the GnRH neuronal network) and the gonads. Hormones released from the hypothalamus, pituitary and gonads (HPG) are key parts of this communication. Patterned, pulsatile release of GnRH from the hypothalamus promotes pituitary release of luteinizing hormone (LH) and follicle-stimulating hormone, which together regulate ovarian function. Gonadal hormones, in turn, provide essential feedback signals to the brain and pituitary to shape GnRH and LH release.
This is a preview of subscription content, access via your institution
Access options
Access Nature and 54 other Nature Portfolio journals
Get Nature+, our best-value online-access subscription
24,99 € / 30 days
cancel any time
Subscribe to this journal
Receive 12 print issues and online access
195,33 € per year
only 16,28 € per issue
Rent or buy this article
Prices vary by article type
Prices may be subject to local taxes which are calculated during checkout
Original articles
Daniels, T. L. & Berga, S. L. Resistance of gonadotropin releasing hormone drive to sex steroid-induced suppression in hyperandrogenic anovulation. J. Clin. Endocrinol. Metab. 82 , 4179–4183 (1997)
CAS PubMed Google Scholar
Pastor, C. L., Griffin-Korf, M. L., Aloi, J. A., Evans, W. S. & Marshall, J. C. Polycystic ovary syndrome: evidence for reduced sensitivity of the gonadotropin-releasing hormone pulse generator to inhibition by estradiol and progesterone. J. Clin. Endocrinol. Metab. 83 , 582–590 (1998)
Related articles
Sullivan, S. D. & Moenter, S. M. Prenatal androgens alter GABAergic drive to gonadotropin-releasing hormone neurons: implications for a common fertility disorder. Proc. Natl Acad. Sci. USA 101 , 7129–7134 (2004)
Article CAS PubMed PubMed Central Google Scholar
Silva, M. S. B. et al. Overactivation of GnRH neurons is sufficient to trigger polycystic ovary syndrome-like traits in female mice. EBioMedicine 97 , 104850 (2023)
Download references
Author information
Authors and affiliations.
Centre for Neuroendocrinology and Department of Physiology, University of Otago School of Biomedical Sciences, Dunedin, New Zealand
Rebecca E. Campbell
You can also search for this author in PubMed Google Scholar
Corresponding author
Correspondence to Rebecca E. Campbell .
Ethics declarations
Competing interests.
The author declares no competing interests.
Rights and permissions
Reprints and permissions
About this article
Cite this article.
Campbell, R.E. Beyond the ovary: rewiring our perspective on polycystic ovary syndrome. Nat Rev Endocrinol 20 , 257 (2024). https://doi.org/10.1038/s41574-024-00963-3
Download citation
Published : 20 February 2024
Issue Date : May 2024
DOI : https://doi.org/10.1038/s41574-024-00963-3
Share this article
Anyone you share the following link with will be able to read this content:
Sorry, a shareable link is not currently available for this article.
Provided by the Springer Nature SharedIt content-sharing initiative
Quick links
- Explore articles by subject
- Guide to authors
- Editorial policies
Sign up for the Nature Briefing newsletter — what matters in science, free to your inbox daily.

Click through the PLOS taxonomy to find articles in your field.
For more information about PLOS Subject Areas, click here .
Loading metrics
Open Access
Peer-reviewed
Research Article
Impact of polycystic ovary syndrome on quality of life of women in correlation to age, basal metabolic index, education and marriage
Roles Investigation
Affiliation Department of Pharmacology, Santosh Medical College, Santosh University, Ghaziabad, Uttar-Pradesh, India
Roles Data curation
Affiliation Department of Gynecology and Obstetrics, All India Institute of Medical Sciences, Patna, Bihar, India
Roles Project administration
* E-mail: [email protected] (KD); [email protected] (MSA)
Roles Formal analysis, Writing – original draft, Writing – review & editing
Affiliation College of Pharmacy, King Khalid University, Abha, Kingdom of Saudi Arabia

- Fauzia Tabassum,
- Chandra Jyoti,
- Hemali Heidi Sinha,
- Kavita Dhar,
- Md Sayeed Akhtar
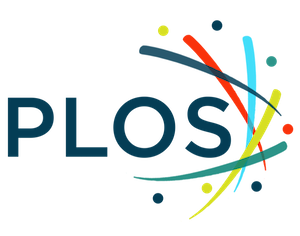
- Published: March 10, 2021
- https://doi.org/10.1371/journal.pone.0247486
- Reader Comments
Polycystic ovary syndrome (PCOS) is the major endocrine related disorder in young age women. Physical appearance, menstrual irregularity as well as infertility are considered as a sole cause of mental distress affecting health-related quality of life (HRQOL). This prospective case-control study was conducted among 100 PCOS and 200 healthy control cases attending tertiary care set up of AIIMS, Patna during year 2017 and 2018. Pre-validated questionnaires like Short Form Health survey-36 were used for evaluating impact of PCOS in women. Multivariate analysis was applied for statistical analysis. In PCOS cases, socioeconomic status was comparable in comparison to healthy control. But, PCOS cases showed significantly decreased HRQOL. The higher age of menarche, irregular/delayed menstrual history, absence of child, were significantly altered in PCOS cases than control. Number of child, frequency of pregnancy, and miscarriage were also observed higher in PCOS cases. Furthermore, in various category of age, BMI, educational status and marital status, significant differences were observed in the different domain of SF-36 between PCOS and healthy control. Altogether, increased BMI, menstrual irregularities, educational status and marital status play a major role in altering HRQOL in PCOS cases and psychological care must be given during patient care.
Citation: Tabassum F, Jyoti C, Sinha HH, Dhar K, Akhtar MS (2021) Impact of polycystic ovary syndrome on quality of life of women in correlation to age, basal metabolic index, education and marriage. PLoS ONE 16(3): e0247486. https://doi.org/10.1371/journal.pone.0247486
Editor: Antonio Simone Laganà, University of Insubria, ITALY
Received: July 24, 2020; Accepted: January 1, 2021; Published: March 10, 2021
Copyright: © 2021 Tabassum et al. This is an open access article distributed under the terms of the Creative Commons Attribution License , which permits unrestricted use, distribution, and reproduction in any medium, provided the original author and source are credited.
Data Availability: Data cannot be shared publicly because of confidentiality. Data are available from the Ethics Committee, AIIMS, Patna for researchers who meet the criteria for access to confidential data. Contact information for the ethics committee: (The Chairman, Institutional review board, All India Institute of Medical Sciences, Patna, Bihar (India), PIN-801507).
Funding: This work is supported by the Dean of Scientific Research, King Khalid University for the financial support is greatly appreciated for the general research Project under grant number [GRP/190/42], awarded to MSA.
Competing interests: The authors have declared that no competing interests exist.
Introduction
Polycystic ovary syndrome (PCOS) is a major endocrine disorder in young age women affecting their health-related quality of life (HRQOL) and their mental well-being as well [ 1 , 2 ]. Moreover, this develop into lifelong health condition that continues far beyond the young ages and affects around 5 million young age population in the United States of America [ 3 , 4 ]. In India, PCOS has been reported to vary between racial counterparts with an estimated prevalence of 9.13% in adolescents [ 5 ]. The major changes in physical appearance, obesity, along with menstrual irregularity have been found to be the main contributing factor of psychological dilemma [ 6 – 8 ]. PCOS negative impact is always underestimated and dominates on women’s life and may lead to a risk for serious anxiety and psychological disorder [ 9 , 10 ]. Importantly, the psychological burden greatly varies with the change in geographical areas and societal perceptions (Barnard et al., 2007; Brady et al., 2009). These patients may experience characteristics of PCOS as stressful and may be at higher risk for depression and anxiety disorders and even this may lead towards suicidal tendency [ 9 , 10 ].
Clinically, PCOS is characterized by either oligoovulation or anovulation and hyperandrogenism that may cause infertility, and other related metabolic disorders [ 11 ]. This progresses to increased risk of reproductive issues like infertility endometrial cancer, gestational as well as mental disturbances [ 12 ]. However, novel treatments and therapies can then be targeted toward improving those problems, which are most important for the individual concerned [ 13 , 14 ]. Recently, increased importance has been given on understanding the impact of PCOS symptoms and in particular about the feminine identity and thus their treatment from the patients’ perspective for the better quality of life (QOL). HRQOL is a self-perceived health status as a consequence of any disease that is measured by health status questionnaires [ 15 ]. Therefore, HRQOL questionnaires like Short Form Health Survey-36 (SF-36) for PCOS, was used to understand the impact of PCOS and evaluating individual patients’ health status and monitoring and comparing disease burden [ 16 , 17 ]. The SF-36 scale leaves out important detrimental issues linked to PCOS patients such as physical and emotional symptoms associated with menses [ 16 ]. PCOS questionnaire has reasonable internal reliability, good test-retest reliability, good concurrent and discriminated validity, and a reasonable factor analysis making PCOS questionnaire a useful and promising tool for HRQOL in PCOS cases.
At present, there is a paucity of information related to PCOS among women of the reproductive age group in India, in particular, North India. Thus, considering these factors into account, this prospective study was planned to compare socioeconomic status (SDS) and association of age, body mass index (BMI), education level and marital status between PCOS and healthy control cases among the women in the reproductive age group visiting the department of gynaecology and obstetrics of tertiary care hospital.
Material and methods
Ethical approval.
Ethical approval (SU/2017/1226-3) was obtained from the institutional review board of Santosh medical college, Uttar Pradesh, India. The institutional review board of All India Institute of Medical Sciences, Patna, India, granted study site approval (176/AIIMS/PAT/IEC/2017). Informed consent form was obtained from parents or guardians of the minors (<18 years).
Study design
This prospective, cross sectional, observational study was designed and conducted in the tertiary care teaching hospital of north India.
Study setting
Patients visiting the outpatients’ department of Gynecology and Obstetrics, All India Institute of Medical Sciences, Patna (India) were included in the study.
Participants
Patients diagnosed with PCOS, based on criteria derived from the 2003 ESHRE/ASRM (Rotterdam criteria) were arbitrarily enrolled in the study. PCOS is diagnosed as the presence of at least two of three of the following: 1) Oligo/anovulation, 2) hyperandrogenism, 3) Polycystic ovaries [ 18 ]. A healthy control (HC) was selected from participants of the same population and having regular menses and had no clinical features of hyperandrogenism as well as infertility.
Data sources
Data was collected after describing both written and verbal information about the study. After explaining, the informed consent form was signed by each participant and then they were requested to complete the questionnaires. Face-to-face interviews were conducted by investigators to the subjects meeting the inclusion criteria and consented for the participation into the study in three parts: Part A: Semi-structured, pre-validated questionnaires were used for collecting information on the socio-demographic, economic and reproductive history. Part B: Pre-validated SF-36 questionnaire is a standard diagnostic tool for evaluating various aspects of the HRQOL over the previous 4 weeks [ 19 ]. Its validity, sensitivity, reliability, internal consistency and stability, as well as test-retest reliability have frequently been confirmed in various studies [ 20 – 22 ]. SF-36 contains 8 domains: general health, physical functioning, and role limitations due to physical health, role limitation due to the emotional problem, body pain, social functioning, energy/fatigue and emotional well-being. The scores for each domain range from 0–100, where higher scores indicate better condition.
The sample size was estimated post assuming α-error of 0.05, power of 80%, percentage of controls having a poor quality of life to be 20% based on previous studies and odds of poor QOL among cases to be twice than among controls. Hence, a total of 100 PCOS cases and 200 healthy control cases were enrolled in the study.
Inclusion criteria
We included all diagnosed case of PCOS only, female from menarche to menopausal age between the age of 10–49 years, and those given informed consent.
Exclusion criteria
Patients having cognitive or developmental disabilities/another major illness that substantially influenced the HRQOL of women, confirmed malignancy and deformities, as well as breastfeeding women were excluded from the study.
Statistical analysis
Data were analyzed by using statistical software-Stata Version 14.0 (Stata Corp, Texas, USA). After checking for the normality condition for continuous variables, the appropriate statistical test was applied. Confounders like excessive body weight were taken into consideration. Quantitative data expressed as mean±SD, minimum and maximum followed normal and skewed distribution respectively. Analysis of covariance model (ANCOVA) was used to address potential confounders. Categorical variables expressed as frequency and percentage. Pearson Chi-Square test and Fisher exact test were used to checking the association between qualitative variables and categorical variables. Logistic regression analysis was used to estimate odds (95% CI) and models were robust for PCOS and other variables. Multivariable linear regression analysis was performed to observe the association between the variables. Independent t-test and One Way ANOVA used to compare normally distributed continuous variable between two and three categories respectively. Rank sum/Kruskal Wallis test used for comparing skewed continuous variables among categories and to look association between demographic categories. For all statistical tests, P-value < 0.05 is considered as statistical significant.
The outcome of socioeconomic status (SES) of a woman with PCOS and HC cases are mentioned in Table 1 . The women with PCOS and HC were comparable in respect of marital status and family type. Statistically significant differences were observed between PCOS and HC in terms of age (P<0.020), BMI (P<0.001), educational status (P<0.001), marital status (P>0.05) and work category (P<0.001). Total 97% of PCOS case was below the age of 30 years in comparison to 78% of control. Among all PCOS cases, 60% was student and almost 54% received higher education. Among the HC group, 39% was student and only 15% received higher education (P<0.001). A higher percentage of PCOS cases (16%) belong to greater BMI (>30) in comparison to HC (2%).
- PPT PowerPoint slide
- PNG larger image
- TIFF original image
https://doi.org/10.1371/journal.pone.0247486.t001
As shown in Table 2 , among the PCOS group, a significant percentage of women (33%) has menarche at age greater than 14 years and there was no any HC cases lies in this category (P<0.001). In respect of menstrual history, PCOS cases have a higher percentage of irregular (45%) and delayed (54%) menses and this comprises a signify`cant difference (P<0.001) in comparison to HC that was 8% irregular and no any delay in menses were observed. Around 64.3% of cases of PCOS women have no child (P<0.001) in contrast to HC cases (9.5%). However, 86.67% PCOS cases have less than ≤ 2 children in comparison to HC where 69.47% have less than ≤2 children (P<0.169). In terms of pregnancy, almost 77.27% PCOS women got pregnant ≤2 times in comparison to HC cases (P>0.05).
https://doi.org/10.1371/journal.pone.0247486.t002
As depicted in Table 3 , Overall differences of mean in PCOS and HC case comparable in respect of BMI (P<0.125).
https://doi.org/10.1371/journal.pone.0247486.t003
However, the highest range of BMI was more in PCOS cases in comparison to HC. Whereas, a statistically, significant differences in mean were observed in respect of age (P<0.009), age at marriage (P<0.001), age of menarche (P<0.001), number of children (P<0.001) and number of pregnancy (P<0.006) between PCOS and HC cases. In case of age, women with PCOS at age ≤19 showed significantly higher score for of general health (P<0.001), role limitation due to physical health (P<0.001), role limitation due to the emotional problem (P<0.022), pain (P<0.025) and social function (P<0.010) in comparison to age >30. However, comparable differences were observed in physical function (P<0.116), energy or fatigue (P<0.087) and emotional well-being (P<0.108). In HC cases, women of age ≤19 showed a statistically higher score in general health (P<0.001), physical health (P<0.001), role limitation due to the emotional problem (P<0.005) and energy/fatigue (P<0.001). Comparable differences were observed for role limitation due to physical health (P<0.818), pain (P<0.424), social functioning (P<0.110) and emotional well-being (P<0.147; Table 4 ).
https://doi.org/10.1371/journal.pone.0247486.t004
As per BMI is concerned, PCOS women scored high and statistically significant differences were observed in case of BMI those who have value <18 in comparison to >30. In addition, significant differences were observed for general health score (P<0.001), physical health (P<0.001), energy and emotion (P<0.001). Whereas, comparable differences observed in role limitation due to physical health (P<0.085), role limitation due to the emotional problem (P<0.565), pain (P<0.189), social function (P<0.549) and emotional well-being (P<0.127). In HC case, there no significant difference was observed in all the eight domains of SF-36 ( Table 5 ). As per the level of education is concerned, HRQOL score was higher in all eight domain of SF-36 in well-educated women in comparison to illiterate or women having education of primary level, but this difference was observed to be statistically non-significant and comparable. In case of HC women, HRQOL score in graduation level was higher and significant differences were observed in relation to the level of education for SF-36 domains like general health (P<0.001), physical health (P<0.039) and energy/fatigue (P<0.003). However, we observed comparable differences among all other domains of SF-36 ( Table 6 ).
https://doi.org/10.1371/journal.pone.0247486.t005
https://doi.org/10.1371/journal.pone.0247486.t006
We observed significant differences between married and unmarried PCOS cases in terms of general health (P<0.001), physical functioning (P<0.027), role limitation due to physical health (P<0.006), role limitations due to emotional problems (P<0.002), pain (P<0.001), social functioning (P<0.001), energy/fatigue (P<0.003) and emotional well-being (P<0.001). Whereas, in HC cases, no differences were observed between married and unmarried cases in regarding SF-36 domain score for role limitation due to physical health (P<0.538), role limitation due to the emotional problem (P<0.105), Pain (P<0.044), social functioning (P<0.225), emotional well-being (P<0.857). However, significant differences were observed for general health (P<0.001), physical health (P<0.002) and energy/fatigue (P<0.001) among married and unmarried HC cases ( Table 7 ).
https://doi.org/10.1371/journal.pone.0247486.t007
The Fig 1 and Table 8 exhibits the regression analysis data plot and we was observed strong association between infertility and menstrual irregularities (P<0.049) as well as emotional well being (P<0.001) of PCOS patients. We also observed infertility (P<0.001) and hirsutism (P<0.05) as a major predictor affecting in all domain scores ( Table 9 ).
https://doi.org/10.1371/journal.pone.0247486.g001
https://doi.org/10.1371/journal.pone.0247486.t008
https://doi.org/10.1371/journal.pone.0247486.t009
PCOS has no any constant treatment due to its multifaceted features. However, lifestyle modification, hormonal contraceptives and some other drugs like inositol, clomiphene, eflornithine, finasteride, flutamide, letrozole, metformin, spironolactone has been reported to ameliorate the PCOS symptoms [Williams T, Mortada R, Porter S. Diagnosis and treatment of polycystic ovary syndrome. American family physician. 2016; 94(2): 106–113; Lagana AS, Garzon S, Casarin J, Franchi M, Ghezzi F. Inositol in Polycystic Ovary Syndrome: Restoring Fertility through a Pathophysiology-Based Approach. Trends Endocrinol Metab. 2018;29(11):768–780; Lagana AS, Garzon S, Unfer V. New clinical targets of d-chiro-inositol: rationale and potential applications. Expert Opin Drug Metab Toxicol. 2020;16(8):703–710].
PCOS is an endocrine disorder and its long term complications affect various aspects of HRQOL in women [ 23 , 24 ]. Despite the various evidence about compromised HRQOL in women with PCOS, we further explored the other determinants that may help the clinician in care of the patient well-being [ 17 , 25 , 26 ]. Overall, we demonstrated the drastically compromised HRQOL in young women suffering from PCOS. As earlier reported, the woman with PCOS belongs to a lesser age group in relation to HC indicating a higher prevalence of PCOS cases in young age woman especially in adolescents [ 27 ]. As per SDS is concerned, the major difference between PCOS and control cases was observed in case of age, BMI and level of education [ 28 ]. Thus, all this indicated that PCOS affects HRQOL more in the young woman and the SDS definitely affect the prevalence of PCOS. The age of menarche in the majority of women was > 18 years as earlier reported [ 29 ]. This was in contrast to other reports [ 30 ]. Consistently, increased age of menarche was observed in PCOS cases indicating the impact of first menstruation in young women life and in the development of PCOS and another reproductive as well as metabolic disorder [ 31 , 32 ].
As previously reported, we observed a direct correlation between the PCOS and irregular or delayed menses and having no children that can be taken as a symptom of PCOS diagnosis [ 33 ]. The higher number of women having lesser than two children and lesser number of times get pregnant further supported this compromised HRQOL [ 30 ]. This compromised HRQOL in PCOS case was further supported by our study.
The overall decreased mean of BMI and age at menarche indicated as PCOS symptoms. Concurrently, mean age, age at marriage, number of children and frequency of pregnancy was less in PCOS cases than control. Consistent to the previous study, these indices corroborate above findings and strongly indicated the deterioration of HRQOL in PCOS women [ 28 ]. Altogether, higher fertility disorder in PCOS cases was observed that directly affects their HRQOL due to physical, social as well as emotional issues.
Furthermore, in PCOS cases, physical, social and emotional well-being more affected as evidenced from all eight domains of SF-36 indicating strongly compromised HRQOL than HC cases [ 34 ]. In particular, we also compare the mean score in relation to age, BMI, educational level and marital status. Consistent with the previous report, increasing the age had a more negative impact on different domains of SF-36 in PCOS cases than HC cases. Comparable scores in PCOS women with increasing age for physical health, energy and emotional well-being may be due to improved regular menses with age concurrent to improved PCOS features and loss of societal fear. Whereas, in HC cases, changes in normal life trend in the prospect of HRQOL was seen with increasing age indicating normal HRQOL [ 35 ].
In a similar fashion, with the increase in the BMI, physical activity was not affected in PCOS cases as observed from different domains of SF-36 but the emotional problem was more affected in PCOS cases in comparison to HC. This may be a major reason for compromised HRQOL in PCOS women [ 36 , 37 ]. In HC cases, none of the scores of SF-36 domains was different between BMI groups. Consistent to previous studies, with increasing the level of education, all the domains of SF-36 in PCOS cases have improved HRQOL. Similar to other HRQOL studies in different diseases, where well-qualified patients have better HRQOL than illiterate cases [ 38 ]. We also observed that well-qualified group probably have higher number of PCOS cases that directly support that improved SDS is a major contributing factor in developing PCOS. The differences in scores of all the domains of SF-36 were observed in married PCOS cases in contrast to unmarried. Thus, consistent to the previous report, the HRQOL of the unmarried cases was better in comparison to married women [ 39 ]. Whereas, in HC cases, all physical, social, as well as emotional wellness, were similar in both married and unmarried women. This may be due to their social independency and quality of education in young women with PCOS.
As reported earlier, infertility and hirsutism emerges as the major problem affecting the overall HRQOL and a strong association has been observed between infertility and emotional well-being [ 40 ].
Our data compares the relations between PCOS and HC cases of overall HRQOL. We explored the strong association between PCOS and SES, and suggest that with increasing age and BMI PCOS patients had lower scores on SF-36; opposite association was with education level. However, Infertility emerges as the major predictor affecting overall HRQOL in PCOS cases. The present study does have its limitations of not measuring biochemical assessment and ultrasonography indices.
Acknowledgments
The authors are thankful to Dean of Scientific Research, King Khalid University and the College of Pharmacy, Department of Clinical Pharmacy for providing facilities to carry out our research work. I also want to acknowledge Dr. R. M. Pandey, Professor & Head, Department of Biostatistics, All India Institute of Medical Sciences, Delhi (India) for supporting me to analyse and interpret my data.
- View Article
- Google Scholar
- PubMed/NCBI
- 3. Centers for disease control and prevention, 2019. Accessed on 06/11/2019. https://www.cdc.gov/diabetes/library/spotlights/pcos.html .
- Open access
- Published: 11 January 2023
Insulin resistance in polycystic ovary syndrome across various tissues: an updated review of pathogenesis, evaluation, and treatment
- Han Zhao 1 ,
- Jiaqi Zhang 1 ,
- Xiangyi Cheng 1 ,
- Xiaozhao Nie 1 &
- Bing He 1
Journal of Ovarian Research volume 16 , Article number: 9 ( 2023 ) Cite this article
19k Accesses
40 Citations
29 Altmetric
Metrics details
Polycystic ovary syndrome (PCOS) is a common endocrine disorder characterized by chronic ovulation dysfunction and overabundance of androgens; it affects 6–20% of women of reproductive age. PCOS involves various pathophysiological factors, and affected women usually have significant insulin resistance (IR), which is a major cause of PCOS. IR and compensatory hyperinsulinaemia have differing pathogeneses in various tissues, and IR varies among different PCOS phenotypes. Genetic and epigenetic changes, hyperandrogenaemia, and obesity aggravate IR. Insulin sensitization drugs are a new treatment modality for PCOS. We searched PubMed, Google Scholar, Elsevier, and UpToDate databases in this review, and focused on the pathogenesis of IR in women with PCOS and the pathophysiology of IR in various tissues. In addition, the review provides a comprehensive overview of the current progress in the efficacy of insulin sensitization therapy in the management of PCOS, providing the latest evidence for the clinical treatment of women with PCOS and IR.
Polycystic ovary syndrome (PCOS) is currently recognized as the most common endocrine disorder in women of reproductive age, with a worldwide prevalence ranging from 6 to 21%, depending on the diagnostic criteria [ 1 , 2 , 3 , 4 , 5 ]. PCOS is a heterogeneous disease characterized by hyperandrogenism, dysfunctional ovulation, and polycystic ovary morphology, accompanied by metabolic abnormalities, such as insulin resistance (IR) and obesity. However, the underlying pathogenesis of PCOS remains unclear. Recent studies have suggested that genetics, epigenetic changes, environmental factors, oxidative stress, chronic low-grade inflammation, mitochondrial dysfunction, and metabolic disorders are involved in PCOS, thus damaging normal ovarian function [ 6 , 7 , 8 , 9 , 10 , 11 , 12 , 13 ]. IR and compensatory hyperinsulinaemia (HI) are considered major drivers of PCOS pathophysiology and are involved in the development of hyperandrogenaemia and reproductive dysfunction by various mechanisms [ 14 ].
IR and compensatory hyperinsulinaemia (HI) are present in 65–95% of women with PCOS, including the vast majority of overweight and obese women and more than half of women of normal weight. IR is independent of and exacerbated by obesity [ 14 , 15 , 16 , 17 , 18 ]. Currently, there are four commonly recognized phenotypes of PCOS: type A, polycystic ovary (PCO), chronic oligo-anovulation (OA) and hyperandrogenism (HA); type B, OA and HA; type C, PCO and HA; and type D, PCO and OA [ 19 ]. IR is present in all phenotypes, and insulin sensitivity varies according to the PCOS phenotype. IR is the most common classical phenotype (Types A and B) (80%), followed by ovulating PCOS (65%) and non-hyperandrogenaemic PCOS (38%) [ 20 ]. Women with PCOS and IR have a significantly increased risk of adverse pregnancy outcomes [ 21 , 22 ] and chronic diseases, such as type 2 diabetes mellitus (T2DM), cardiovascular disease, and metabolic syndrome, which seriously affect the physical and mental health of women of childbearing age, increasing their social burden [ 23 , 24 , 25 ]. However, the root cause of IR in PCOS is largely unknown and the underlying mechanism remains to be elucidated. Therefore, recognizing the strong influence of IR on the occurrence and development of PCOS, accurate assessment of insulin sensitivity in the early stages of PCOS, and effective intervention on IR are essential to reduce the risk of long-term complications. Lifestyle change is the treatment of choice for all women with PCOS, and insulin sensitization is a promising choice for the chronic treatment of women with PCOS. This paper aims to summarize recent findings on the involvement of IR in the occurrence and development of PCOS and the mechanism of IR in various tissues. Furthermore, we aim to summarize and provide an update on the current research status of insulin sensitization therapy for women with PCOS to provide more effective and reasonable clinical treatment.
An extensive literature search was performed up to July 2022 in PubMed, Google Scholar, Elsevier, and UpToDate databases. Keywords and subject terms included (“PCOS” AND “insulin”) OR (“PCOS” AND “insulin” AND “tissues”) OR (“PCOS” AND “insulin” AND “pathogenesis”) OR (“PCOS” AND “insulin” AND “diagnosis”) OR (“PCOS” AND “insulin” AND “evaluation”) OR (“PCOS” AND “insulin” AND “therapy”). Only English-language research papers were considered. In addition, publications focus on the new ones (since 2018) and exclude those without full manuscripts.
Pathogenesis of insulin resistance in polycystic ovary syndrome
Genetics and foetal origin.
PCOS is an autosomal dominant genetic disease with various expression patterns that begins in early life, and metabolic changes precede reproductive abnormalities. A clustering analysis of 893 women with PCOS identified the metabolic subtype of PCOS, which was characterized by higher BMI and glucose and insulin levels with relatively low SHBG and LH levels [ 5 ]. IR is one of the prominent phenotypic characteristics of PCOS [ 26 ]. Twin and family cluster studies have suggested HI has a genetic component in PCOS, and a family history of T2DM is associated with significant insulin secretion defects [ 27 , 28 ]. The daughters of women with PCOS develop HI and lower adiponectin levels before puberty [ 29 ], which persist throughout adolescence [ 30 ].
PCOS is associated with specific gene mutations, and most gene variants identified in genome-wide association studies are involved in regulating sheath steroid production, follicular maturation, or insulin signalling through the modified proteins they encode, such as insulin receptors, LH/HCG receptor activators, cell traffic proteins, and transcription factors [ 31 , 32 ]. Genome-wide association studies on European, Chinese, and Indian populations have established that some insulin receptor (INSR) gene variants (rs2059807 and rs1799817) are significantly associated with IR in women with PCOS [ 33 , 34 ]. Studies of Indian women suggest that C/T polymorphisms in the INSR tyrosine kinase domain may be susceptible variants in women with normal-weight PCOS, contributing to the development of IR and compensatory HI [ 35 ]. A meta-analysis showed that the Gly972Arg polymorphism in insulin receptor substrate 1 (IRS-1) mediates the pathogenesis of PCOS by increasing fasting glucose levels and is a risk factor for susceptibility to PCOS [ 36 , 37 ]. However, the genetic assessment of insulin-related genes is affected by the diagnostic criteria and genotyping methods employed with patients, resulting in different results [ 38 ]. Exposure to adverse intrauterine environments can lead to varying degrees of IR and HI. Exposure to dihydrotestosterone and insulin in the second trimester of pregnancy produces a PCOS-like phenotype and increases the risk of miscarriage [ 39 ]. Intrauterine growth restriction can affect foetal insulin secretion, and insulin resistance trends in PCOS may be involved in developmental origin and preprogramming as a nutritional compensation mechanism [ 40 , 41 ]. Adolescents and young women with a history of low birth weight are more likely than normal women to have PCOS-like manifestations of IR and high androgen levels [ 42 , 43 ].
- Insulin signal transduction pathway
Insulin is a small peptide receptor-binding hormone released by pancreatic beta cells, which binds to cell surface receptors. INSR is a heterotetramer composed of α- and β-subunits linked by disulphide bonds. The extracellular α-subunit is responsible for binding to the ligands. The β-subunit is a glycoprotein spanning the cell membrane with tyrosine kinase activity [ 44 ]. Insulin binding to receptors induces specific tyrosine autophosphorylation, which phosphorylates intracellular substrates, including IRS1-4, SRC homologues, and collagen homologues (Shc), leading to a complex intracellular cascade that initiates insulin signal transduction [ 45 ]. Insulin has two main signalling pathways: metabolism and mitosis. Metabolism is primarily mediated through phosphatidylinositol 3-kinase (PI3-K) and serine/threonine kinase Akt/protein kinase B (PKB), also known as the PI3-K pathway. Through these pathways, insulin stimulates glucose uptake by promoting the translocation of glucose transporter 4 (GLUT4) from intracellular vesicles to the cell surface [ 46 ] and leads to the inactivation of serine phosphorylation of glycogen synthase kinase 3 (GSK3), increasing glycogen, fatty acid, and protein synthesis. It also activates mammalian target of rapamycin (mTOR) to regulate protein synthesis and degradation [ 46 ]. The mitotic pathway is the mitogen-activated protein kinase-extracellular signal-regulated kinase (MAPK-ERK) pathway, which is activated by insulin receptor-mediated phosphorylation of Shc or IRS. This progressively stimulates the translocation of cascade erk1/2 to the nucleus and phosphorylates transcription factors to stimulate cell growth and differentiation and regulate gene expression [ 47 , 48 ]. Increased serine phosphorylation and decreased tyrosine phosphorylation of insulin receptors and IRS can terminate insulin action, resulting in post-binding defects in insulin signal transduction and leading to insulin dysfunction in women with PCOS [ 49 , 50 ].
Hyperinsulinaemia and tissue insulin resistance
IR in PCOS is caused by impaired insulin action in various target tissues, which is characterized by basal compensatory HI and a reduced insulin response to glucose overload. PCOS affects the majority of organ systems and tissues. Insulin plays different roles in different tissues in balancing the supply and demand of nutrients. HI caused by tissue IR is central to PCOS pathology [ 51 ]. IR in women with PCOS selectively and mutually affects metabolism or mitotic pathways in classical insulin target tissues (e.g., liver, skeletal muscle, and adipose tissue) and non-classical insulin target tissues (e.g., ovary, pituitary gland) [ 52 ]. In addition, androgen overload, lipid deposition, inflammatory cytokines, and other systemic factors are also involved in the IR process of peripheral tissues [ 53 ] (Fig. 1 ).
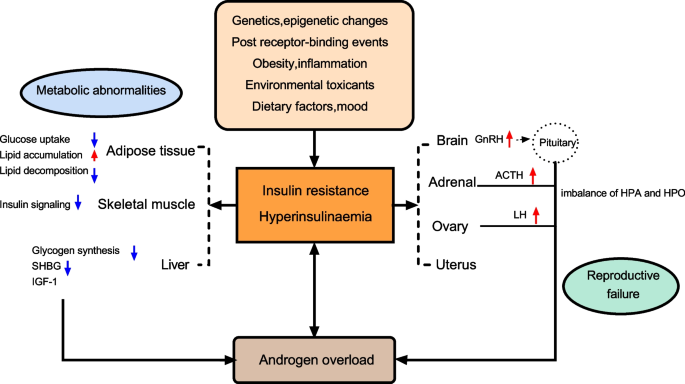
A summary of the most representative impact of IR and HI in women with PCOS. Abbreviations: SHBG: sex hormone-binding globulin; LH: luteinizing hormone; IGF1: insulin growth factor 1; GnRH: gonadotropin-releasing hormone; ACTH: adrenocorticotropic hormone; HPO: Hypothalamus-pituitary-ovary; HPA: Hypothalamus–pituitary–adrenal
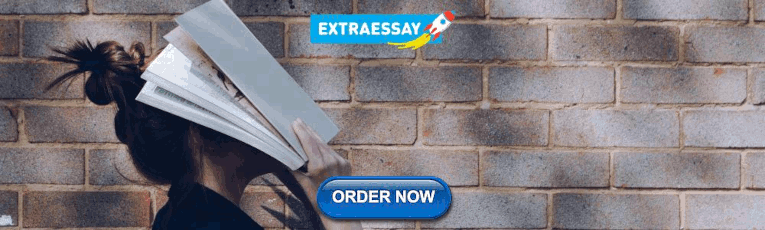
Adipose tissue
Adipose tissue plays a central role in systemic glucose metabolism and insulin sensitivity. Compared with body mass index (BMI)-matched control women, women with PCOS showed systemic fat accumulation and significantly increased subcutaneous fat cell volume, whereas visceral fat volume was only increased in PCOS phenotype A [ 54 , 55 , 56 ]. The increase in adipose tissue volume, especially visceral adipose tissue volume, is closely associated with whole-body IR [ 57 ]. The IR of adipose tissue results in decreased glucose uptake and lipid accumulation, and significantly inhibits lipid decomposition. Excessive fatty acids flow into skeletal muscle and liver, resulting in lipid storage and aggravating IR of skeletal muscle and the liver [ 58 ].
Adipose tissue IR in women with PCOS is influenced by circulating androgen levels and excessive energy intake. Androgens induce adipocyte IR by affecting the phosphorylation of insulin-stimulated protein kinase C (PKC), leading to a decrease in insulin-induced GLUT-4 content in PCOS abdominal subcutaneous adipocytes and a decrease in insulin-stimulated serine phosphorylation of GSK3, indicating the presence of insulin receptor binding or phosphorylation defects in adipocyte IR [ 59 ]. This impairs the effect of insulin on glucose metabolism but does not affect insulin-induced mitosis [ 59 ]. Androgens also regulate lipid metabolism and adipocyte differentiation, and induce the accumulation of abdominal adipose tissue. Animal models have shown that prenatal and postnatal exposure to androgens can lead to enlargement of adipocytes, accumulation of visceral fat, and decreased insulin sensitivity in women [ 60 , 61 ]. In addition, testosterone is specific to catecholamine-stimulated lipolysis and can reduce the sensitivity of human subcutaneous fat cells but not visceral fat cells [ 62 ].
Type 1C3 aldosterone reductase (AKR1C3) is the only enzyme expressed in adipose tissue that can convert androstenedione into testosterone, which is widely expressed in the adipose tissue of patients with PCOS [ 63 ]. Studies have shown that AKR1C3 is the main driver of active androgen production in PCOS adipose tissue [ 63 ]. In addition, AKR1C3 expression is regulated by insulin [ 64 ], suggesting a significant correlation between testosterone and circulating insulin levels in adipose tissue. Increased androgen production in adipose tissue and subsequent lipid accumulation and fat mass can lead to systemic IR and lipotoxic organ damage in patients with PCOS [ 63 ]. HI further exacerbates hyperandrogenaemia, resulting in a vicious cycle that exacerbates poor metabolic performance [ 63 ]. In addition, free testosterone seen in PCOS is inversely proportional to the serum level of high-molecular-weight adiponectin, a collagen-like protein synthesized only by adipose cells, which has insulin sensitization and anti-inflammatory effects and decreases with adipose tissue volume [ 65 ]. Decreased adiponectin levels result in decreased PKC activity and insulin signalling [ 66 ]. Adiponectin also stimulates the secretion of hepatic sex hormone-binding globulin (SHBG), suggesting that the effect of androgen’on adipose IR may be influenced by hepatic SHBG through serum free testosterone levels [ 66 ]. Increased adipose tissue and its dysfunction may exacerbate physiological factors and cytokine levels, such as leptin, interleukin 6, and tumour necrosis factor alpha, thereby promoting low-level inflammation, interfering with insulin signalling, causing adipose tissue to release free fatty acids, increasing ectopic fat deposition, and aggravating IR on one’s own and other organizations [ 67 , 68 , 69 ].
Skeletal muscle
Skeletal muscle is responsible for most peripheral glucose uptake regulation, and almost two thirds of the glucose intake after meals is absorbed by skeletal muscle through insulin-dependent mechanisms, making it the most important insulin-resistant tissue [ 70 ]. Muscle insulin-mediated glucose processing, as measured by a normal glucose clamp, was significantly reduced in all women with PCOS compared to women without PCOS [ 71 ]. Skeletal muscle IR in women with PCOS is reflected by impaired insulin-stimulated glucose processing, which is a major risk factor for T2DM in women with PCOS [ 51 ]. However, human studies have not yet determined the molecular mechanism of PCOS-specific IR in skeletal muscle, and there have been many conflicting findings. The potential mechanisms currently considered include genetic and epigenetic programming, signalling pathway and mitochondrial dysfunction, intracellular and extracellular lipid accumulation, and organ system crosstalk [ 72 ].
Initial studies of muscle tissue and cultured myoducts and fibroblasts [ 73 , 74 ] found elevated phosphorylation of serine residues on IRS1/2, resulting in the translocation of GLUT4 and reduced glucose uptake, suggesting defective signalling at the proximal insulin site. Skeletal muscle biopsies of women with PCOS revealed a significant decrease in insulin-mediated IRS1-related PI3-K activation, with an increase in IRS2 abundance as a compensatory change after targeted IRS1 destruction. Subsequent skeletal muscle studies showed decreased phosphorylation of Akt/PKB and the Akt substrate 160-KDA (AS160) [ 75 ]. These studies in obese women with PCOS suggest that possible PCOS-specific defect in insulin signaling of skeletal muscle is proximal and distal to IRS1/2. Hansen et al. studied molecular mechanisms in skeletal muscle underlying IR in normal-weight women with PCOS, finding that decreased insulin sensitivity may only play a role in skeletal muscle IR through AMPK and is associated with low circulating adiponectin levels [ 76 ]. In addition, a lack of insulin-stimulated pyruvate dehydrogenase activation in skeletal muscle may lead to reduced systemic metabolic flexibility and mediation of IR through metabolic signalling pathways [ 76 ]. Furthermore, constitutive activation of the mitotic signal MAPK-ERK1/2 has also been found in skeletal muscle biopsies of women with PCOS, which promotes serine phosphorylation of IRS1 and reduces metabolic signalling in PCOS myotube [ 77 ], suggesting that IR may impact both the metabolic and mitotic pathways in skeletal muscle of women with PCOS.
Animal studies have shown that hyperandrogenaemia promotes IR by increasing serine phosphorylation of Akt/PKB, mTOR ribosomal S6 kinase, and IRS1 in myotubes and promoting visceral fat accumulation [ 78 ]. In addition, hyperandrogenaemia may increase inflammation by activating nuclear factor kappa B (NF-κB), which in turn affects intracellular enzyme pathways associated with insulin receptors [ 51 ]. A recent meta-analysis suggested that obesity, but not HA or IR, appears to predict skeletal muscle mass in reproductive-aged women with PCOS [ 79 ]. Intramuscular lipid accumulation within muscle cells or between fibres may account for a small percentage of skeletal muscle IR [ 80 ]. PCOS is associated with abnormal skeletal muscle gene expression, and it is affected by specific changes in DNA methylation [ 72 ]. Furthermore, a link between TGF-β superfamily ligand signalling and extracellular matrix deposition in PCOS-specific IR results in inappropriate crossing between these cells and their host organs, suggesting that epigenetic as well as tissue crossing is involved in skeletal muscle metabolic abnormalities [ 72 ]. Mitochondrial oxidative phosphorylation genes are downregulated in the skeletal muscle of women with PCOS, suggesting that mitochondrial dysfunction is involved in PCOS-specific IR formation [ 72 , 81 ].
Liver tissue
The liver is also the main site of glucose uptake and storage, accounting for one third of postprandial glucose processing, and the main site of insulin clearance [ 81 ]. Insulin in the liver promotes glycogen synthesis and de novo lipogenesis while also inhibiting gluconeogenesis. PCOS-related hepatic IR is usually only present in obese women, leading to a deficiency in insulin-stimulated liver glycogen synthesis and insulin-mediated inhibition of hepatic glucose production [ 82 ]. IR and compensatory HI can directly inhibit the synthesis of liver SHBG and insulin growth factor 1 (IGF1) binding protein. The former is a glycoprotein synthesized mainly in the liver, and its reduced synthesis leads to increased free testosterone levels [ 83 ]. The reduction of the latter increases the circulating concentration of IGF1, which not only triggers the ovarian membrane cells to produce more androgens, but also reduces specific microRNAs, thus accelerating the apoptosis of granulosa cells and inhibiting follicular development. These two effects together lead to hyperandrogenaemia and follicular development disorders in PCOS [ 13 ].
Insulin in the liver can directly regulate glucose and lipid metabolism and can also be indirectly regulated by fat and muscle insulin action. Direct effects activate de novo lipogenesis, convert excess carbohydrate substrates to triglycerides, and promote liver triglyceride delivery to adipose tissue [ 82 ]. Indirect effects are mainly caused by insulin-mediated inhibition of lipolysis in adipose tissue, which leads to an increase in circulating plasma non-fatty acids in the liver and promotes fat deposition in the liver. Approximately 59% of the lipids in hepatocytes are derived from non-fatty acids produced by adipolysis [ 82 ]. The liver-specific insulin receptor knockout (LIRKO) mouse model suggests that the insulin signalling is essential for the regulation of glucose homoeostasis in the liver and maintenance of normal liver function [ 84 ]; further, it is also a prerequisite for the indirect regulation of adipose insulin. LIRKO mice still showed obvious IR, severe glucose intolerance, and resistance to insulin’s ability to inhibit liver sugar production under the premise of normal insulin signal transduction in fat and muscle tissues [ 82 ]. In addition, hepatic lipid accumulation activates diacylglycerol/PKC and inhibits insulin receptors, affecting insulin signalling and subsequent gluconeogenesis, thereby exacerbating hepatic IR [ 53 ].
In vitro and in vivo studies of liver-specific androgen receptor (AR) gene knockout have found that AR signalling in liver cells mediates hepatic IR in hyperandrogenaemia-induced female mice through a cascade of changes in hepatic insulin signalling and phosphorylation, suggesting that androgens are involved in hepatic IR [ 85 ]. Long-term androgen excess can induce hepatic insulin resistance and steatosis in PCOS-like rats. Under hepatic IR, excessive androgens can promote the development of non-alcoholic fatty liver disease (NAFLD) through apoptosis and autophagy in the liver mitochondria [ 86 ]. NAFLD, a metabolic syndrome characterized by abnormal fat accumulation, is now considered the most common chronic liver disease in the United States, and its prevalence in PCOS has increased significantly in recent years [ 87 ]. Several human studies have shown a close association between NAFLD and liver IR, and IR is an important risk factor for NAFLD in PCOS [ 88 , 89 , 90 ].
The ovaries and uterus
Ovarian androgen overload is the core of PCOS. HI enhances intrathecal steroid production and leads to impaired follicular maturation. Insulin receptors are widely distributed in stromal and follicular ovarian cells, and there is considerable evidence for the direct ovarian effect of insulin on steroid production and the importance of insulin-signalling pathways in ovulation control [ 91 , 92 ]. Under physiological conditions, insulin acts as a helper gonadotropin through its homologous receptor to increase LH-induced androgen synthesis in membrane cells, and LH induces luteinization in granulosa cells [ 93 ]. HI can lead to androgen-dependent anovulation via different mechanisms. Membrane cells are the main site of androgen production in the ovaries. Insulin acts on the membrane cells of the ovary to directly trigger androgen synthesis by increasing the activity of cytochrome P450c17α, a key enzyme that regulates androgen biosynthesis encoded by CYP17. Insulin can also cooperate with LH. The 17α-hydroxylase activity of P450c17 is activated by PI3-K signalling to induce androgen synthesis in membrane cells [ 94 , 95 , 96 , 97 ]. The membrane cells of women with PCOS are more sensitive to the hyperandrogenic effects of insulin than healthy women [ 51 ]. In anovulatory PCOS granulosa cells, the synergistic effect of high insulin and LH levels may induce premature expression of LH receptors in small follicular subsets, leading to premature differentiation of granulosa cells and follicular growth stagnation [ 98 ]. The effect of insulin on glucose metabolism was significantly reduced in granular lutein cells isolated from the ovaries of women with typical PCOS phenotypes, whereas the effect of insulin on steroid production was unchanged [ 98 ]. Reduced phosphorylation of MEk1/2 and MApK-ERk1/2 in PCOS was associated with increased P450c17 expression compared with that in normal membrane cells, contrary to the findings of increased phosphorylation of MEk1/2 and MApK-ERk1/2 in PCOS skeletal muscle [ 74 ]. These results suggest the existence of selective insulin resistance in PCOS ovarian tissue.
Energy metabolism is also critical for normal endometrial function, and endometrial studies of patients with PCOS have shown that IR and HI also negatively affect endometrial physiology. Endometrial tissues express molecules involved in insulin signalling pathways, and the expression of insulin receptors, IRS proteins, AS160, PKC, and GLUT4 in the endometrium of women with PCOS is impaired and associated with adverse reproductive outcomes [ 39 ]. Hyperinsulinaemia can impair decidualization of endometrial stromal cells in vitro through the transcriptional inhibition of FOXO-1 [ 99 ]. In addition, hyperandrogenaemia plays a role in the insulin signalling pathway of the endometrium, reducing the expression of INRS-1 and GLUT-4 in endometrial glandular epithelial cells [ 100 ]. The insulin sensitizer metformin promotes GLUT4 transcription by increasing AMPK, improves IR, and indirectly restores endometrial function in PCOS patients [ 101 ].
Central nervous system
Insulin affects the hypothalamic-pituitary system and can increase the frequency and amplitude of gonadotropin-releasing hormone (GnRH) release pulses through MAPK and increase GnRH gene expression, thereby increasing LH release, enhancing androgen biosynthesis in the ovary, and impairing ovarian function [ 102 ]. Insulin signalling in the central nervous system plays an important role in normal reproduction and body weight regulation. Female mice with neuron-specific destruction of IR genes show increased food intake, disrupted LH release, and impaired ovarian follicle maturation [ 103 , 104 ]. Leptin, one of the earliest known adipokines, is essential in the hypothalamus for maintaining normal body weight and insulin sensitivity [ 105 ]. Specific knockout of insulin receptors and leptin receptors in hypothalamic proopiomelanocortin neurons induces a PCOS phenotype [ 106 ]. The pituitary gland is one of the most important components of the PCOS insulin. Insulin can directly stimulate LH secretion, leading to abnormal reproductive function in PCOS patients [ 107 ]. In addition, IR- and HI-mediated reduction of pituitary sensitivity to GnRH and disruption of pituitary insulin receptors can lead to anovulation [ 108 ]. Women with PCOS had a higher ACTH response to corticotropin-releasing hormone (CRH) stimulation than women who ovulate normally, which was strongly associated with HI severity [ 109 ]. IR and compensatory HI can increase adrenal androgen levels and aggravate PCOS hyperandrogenaemia by increasing adrenal sensitivity to ACTH [ 110 ].
Women with PCOS show hyperactivation of the AR in the preoptic area, hypothalamus, and other limbic structures [ 110 ]. Animal studies have found that male mice with neuronal AR deletions exhibit hypothalamic IR, suggesting that androgens may also promote IR by acting on the central nervous system [ 111 ]. In addition, increased leptin expression in the hypothalamus can aggravate obesity, and enhanced leptin secretion by adipocytes can further contribute to the induction of IR [ 48 ]. the two interact to cause metabolic disorders in PCOS.
Factors affecting insulin resistance in polycystic ovary syndrome
Epigenetic changes (DNA methylation, histone status, and miRNA expression) are involved in the regulation of IR in women with PCOS. A study identified 79 differentially methylated genes in women who have PCOS with or without IR [ 112 ], and hypermethylation of the LAMIN gene promoter was associated with IR in PCOS [ 113 ]. MicroRNAs (miRNAs) are small non-coding RNA involved in the post-transcriptional regulation of genes. As regulators of gene expression, miRNAs are essential genes involved in the control of androgen synthesis, inflammation, adipogenesis, and signalling [ 114 ]. There are significant differences in miRNA expression levels between women with PCOS and healthy women [ 114 ]. Studies have shown that microRNAs secreted into the circulation by adipocyte exosomes and adipose tissue macrophages affect the PI3K/Akt-GLUT4 signalling pathway [ 115 ]. Mir-155-5p and related target genes of PCOS are concentrated in the insulin-sensitive pathway of the ovary, and Mir-222 is also positively correlated with serum insulin levels, suggesting their potential value as biomarkers of PCOS [ 115 ].
Insulin sensitivity can also be negatively affected by changes in diet, the environment, and mood. Recent studies suggest that an imbalance of intestinal flora and abnormal levels of metabolites produced by bacteria in individuals with may lead to insulin receptor signalling deficiency, leading to IR by causing immune system dysfunction, the development of chronic low-grade inflammation, and the enhancement of proinflammatory cytokine synthesis [ 116 ]. Vitamin D deficiency can also affect insulin signalling in tissues by affecting intracellular calcium regulation and exacerbating inflammatory responses [ 117 , 118 ]. Melatonin is involved in regulating insulin secretion, and decreased melatonin secretion at night is associated with increased IR [ 119 ]. In addition, melatonin’s action is mediated by the melatonin receptor (MTNR), and activation of the MTNR1B signalling pathway in pancreatic beta cells reduces insulin secretion [ 120 , 121 , 122 ]. A meta-analysis also showed that MTNR1B RS1083096 and RS2119882 are involved in the pathogenesis of IR in Chinese women with PCOS [ 123 ]. Advanced glycation end products alter cellular translocation of insulin intracellular signalling and glucose transporters in PCOS through a variety of mechanisms, leading to tissue IR [ 124 ]. The endocrine disruptor bisphenol A also disrupts glucolipid metabolism and induces IR in PCOS by altering insulin secretion, adipocyte differentiation, and adipokine secretion [ 125 ]. In addition, chronic stress can trigger the release of cortisol from the hypothalamic–pituitary–adrenal axis, which can stimulate visceral fat accumulation, gluconeogenesis, and lipolysis, leading to IR [ 126 , 127 ].
Diagnosis and evaluation of insulin resistance in polycystic ovary syndrome
The glucose clamp technique is the ‘gold standard’ for evaluating metabolic insulin resistance in vivo. The amount of glucose injected in the steady state was equal to the amount of glucose absorbed by the peripheral tissue, which can be used to measure peripheral sensitivity to insulin [ 128 ]. Minimal model analysis using a frequently sampled intravenous glucose tolerance test (FSIGT) is an alternative to the simplified clamp procedure for assessing insulin secretion in insulin sensitivity experiments [ 129 ]. However, both the clamp test and FSIGT are complex, time-consuming, and expensive sampling procedures that are unsuitable for clinical practice. In recent years, clinical practice has developed many simple, cheap, and effective alternative quantitative indicators, such as BMI, waist circumference, waist-to-hip ratio, wrist circumference [ 130 ]and other anthropometric markers; fasting insulin, oral glucose tolerance test (OGTT), glucose/insulin ratio (G/I), homoeostasis model assessment of insulin resistance (HOMA-IR), quantitative insulin sensitivity test index (QUICKI) [ 131 ], lipid/lipoprotein ratio [ 132 , 133 , 134 ]and other biomarkers. These indices are reasonably correlated with each other and with the gold standard clamp technique. HOMA-IR is currently the best and most widely validated marker, but the cut-off point for the diagnosis of PCOS-IR is still not universally accepted [ 135 ]. Studies suggest that a more complex evaluation of the decrease in insulin sensitivity as a continuous variable is required in clinical practice [ 10 ]. In addition, owing to the strong association between inflammation and IR, inflammatory markers such as interleukin-6 (IL-6) [ 136 ] and ferritin [ 137 ] are becoming increasingly popular in the evaluation of IR, while cytokines such as leptin [ 138 ] and adiponectin [ 139 ] have also been proposed as new IR markers. However, conflicting data limit their use in clinical settings, and more studies are needed to clarify their suitability as IR markers in patients with PCOS [ 140 ].
Treatment of insulin resistance in polycystic ovary syndrome
Lifestyle change.
Guidelines recommend that once women are diagnosed with PCOS and have decreased insulin sensitivity, they should make lifestyle changes and start insulin sensitivity treatment immediately, even if there are no significant changes in glucose tolerance [ 141 ]. The first step in managing IR is lifestyle change, which is the cornerstone of improving multiple endocrine and metabolic disorders in women with PCOS [ 142 ] and can be achieved through appropriate diet and exercise recommendations [ 143 ]. Studies on the relationship between caloric intake and expenditure in women with PCOS have been inconsistent, with preliminary data suggesting that the diets of women with PCOS tend to be high in carbohydrates and fat [ 144 ], with decreased satiety and increased sweet cravings [ 145 ]. Calorie-restricted diets may be the best option for reducing IR and improving body composition [ 146 ]. Studies have shown that the Mediterranean diet—which emphasizes a high intake of vegetables, fruits, seafood, legumes, and nuts; whole grains as staple foods; and the promotion of vegetable oils—combined with a low-carbohydrate regimen improves endocrine disorders and menstrual cycles in overweight patients with PCOS [ 147 ]. International evidence-based guidelines recommend that all women with PCOS, especially those who are overweight or obese, engage in at least 150 min of aerobic exercise per week, including more than 90 min of vigorous exercise [ 148 ]. There have been conflicting conclusions regarding the efficacy of the choice of optimal exercise mode in improving insulin sensitivity in women with PCOS. The heterogeneity of PCOS necessitates individualization of treatment options, and it appears that exercise combined with additional dietary/drug intervention is better for improving insulin sensitivity than either intervention alone [ 149 , 150 , 151 ].
Sleep deprivation is associated with an increased risk of IR, obesity, and T2DM in women with PCOS; therefore, sleep management should also be part of lifestyle change in women with PCOS [ 152 ]. Ensuring adequate, high-quality sleep can be an important initial change in women with PCOS. Since IR is strongly and independently associated with depression in PCOS, lifestyle interventions should be supported by mental health professionals who can provide appropriate psychological care for women with PCOS [ 25 ]. PCOS treatment is a long-term process, and diet and physical exercise require high self-discipline, are time-consuming, and are prone to relapse. Targeting IR is an effective strategy for treating PCOS. Clinical and experimental studies in recent years have explored several promising new therapies for improving IR in women with PCOS. Common pharmacological approaches for reducing IR in women with PCOS are outlined in the chart below (Table 1 ).
- Insulin sensitization therapy
Metformin, the most widely used insulin sensitizer for PCOS, reduces hepatic glucose production, inhibits gluconeogenesis and adipogenesis, improves peripheral tissue sensitivity to insulin, and prevents excessive insulin activity in the ovary [ 153 ]. Numerous studies have shown that metformin not only reduces weight and metabolic disorders but also corrects menstrual patterns, restores ovulation, and even improves chances of pregnancy [ 154 ]. Evidence-based guidelines recommend the use of metformin in obese, insulin-resistant women with PCOS to manage weight and endocrine and metabolic disorders, in conjunction with lifestyle adjustments [ 155 , 156 ]. Metformin improves insulin sensitivity, alleviates metabolic disorders, and ameliorates polycystic symptoms in mice with PCOS by increasing the translocation of the glucose transporters GLUT1 and GLUT4 to cell membranes [ 157 ], activating the AMPK signalling pathway [ 158 ], and reconstructing the role of endogenous insulin-sensitizing molecules, such as adiponectin, in endometrial tissues under pathological conditions [ 159 ]. However, metformin use may be limited by gastrointestinal side effects [ 160 ].
Thiazolidinediones
Thiazolidinediones (TZDs) and peroxisome proliferator-activated receptor γ (PPAR-γ) agonists are true insulin sensitizers. PPAR-γ is a nuclear receptor that enhances insulin activity through a post-insulin receptor mechanism, primarily by improving insulin sensitivity in the adipose tissue and skeletal muscle [ 213 ]. TZDs can be used as alternative drug therapy for PCOS-related metabolic and reproductive abnormalities in women who cannot tolerate or respond poorly to metformin [ 161 , 162 , 213 ]. TZDs are effective as treatments for HI and IR in both lean and obese women with PCOS as well as in improving abnormal glucose tolerance, hyperandrogenaemia, and ovulation disorders in women with PCOS. Several studies and our mesh meta-analysis suggest that TZDs improve IR and dyslipidaemia in PCOS more than metformin does [ 163 , 214 , 215 ]. In addition, the combination of metformin and TZDs has a synergistic effect in the treatment of women with PCOS, conferring greater improvement in IR and menstrual frequency in PCOS than metformin alone [ 215 ]. Women with dyslipidaemia and PCOS may also consider rosiglitazone alone or in combination with low-dose metformin and lifestyle changes [ 119 ]. Compared to trioglitazone and rosiglitazone, pioglitazone shows a higher affinity for the specific receptor PPAR-γ, has more effective insulin sensitization and lower hepatotoxicity, but does not promote weight loss [ 109 ].
New antidiabetic drugs
Many new antidiabetic drugs have shown positive effects in the treatment of PCOS. Glucagon-like peptide-1 analogues (GLP-1RAs) mimic the incretin secreted by the distal small intestine, bind to insulin receptors on beta cells, stimulate insulin secretion, reduce glucagon secretion, inhibit hunger centres, and delay gastric emptying. They also exhibit anti-inflammatory properties [ 216 , 217 ]. Recent studies have shown that GLP-1RAs therapy has excellent therapeutic effects in improving hyperandrogenaemia, increasing menstrual frequency, reducing manifestations of metabolic disorders such as obesity and IR, and reducing long-term cardiovascular risk in obese women with PCOS [ 164 , 166 ]. The combination of GLP-1RAs and metformin appears to be superior to any single agent in reducing body weight, hyperandrogenaemia, IR, and ovulation disorders in women with PCOS and may even improve metabolic outcomes in women who previously had an inadequate response to metformin [ 162 , 217 ]. However, most GLP-1RAs are administered subcutaneously. Simultaneously, correct administration of medication is crucial to reduce the occurrence of adverse reactions [ 164 , 165 , 167 , 168 , 218 ]. Sitagliptin, a DPP-4 inhibitor, enhances early insulin secretion by reducing incretin and GLP-1 degradation, reduces peak glucose after oral glucose intake in overweight women with PCOS, and improves visceral obesity [ 169 ].
Sodium-glucose cotransporter type 1 and type 2 inhibitors (SGLT1/2is) play important roles in glucose homoeostasis by reducing HI and improving IR by acting on glucose (heavy) absorption in the gut and kidney, respectively [ 219 ]. Although the mechanism of action of SGLT1/2is in PCOS has not been fully investigated, weight loss and HI, improved IR and glucose metabolism, and cardioprotective effects are beneficial in PCOS, suggesting that SGLT1/2is may be a novel treatment option [ 170 , 171 , 172 ]. In clinical trials, the SGLT2 inhibitor canagliflozin was not inferior to metformin in reducing HOMA-IR, and canagliflozin also significantly improved menstruation and body weight and reduced triglyceride levels, suggesting that it should be considered an effective treatment for patients with PCOS and IR [ 173 ]. Urinary symptoms are major adverse events associated with SGLT2 inhibitors, and more large-scale randomized controlled trials are expected to be published in the future to explore their therapeutic potential for PCOS [ 220 ].
Natural molecules and dietary supplements
Inositol is a compound with nine forms (sugar alcohols), of which inositol (MI) and d -chiral inositol (DCI) are the most abundant forms present in humans, playing important biological roles in mediating various effects of insulin. Several scientific studies have confirmed that it has excellent insulin sensitization efficiency in women with PCOS and promotes ovulation [ 180 ]. Given that inositol administration is safe and effective in ameliorating the reproduction and metabolism of patients with PCOS, it may be used not only as a treatment for infertile women but also as a preventive treatment during pregnancy [ 180 , 181 , 182 , 183 ]. Appropriate application of MI and a suitable proportion of DCI improves the health of organs and tissues, while long-term high-dose DCI monotherapy in women with PCOS has a negative impact [ 184 ]. Therefore, inositol treatment should be evaluated according to the specific situation and needs of patients, while its optimal ratio still needs to be further clarified and supported by large-scale clinical trials and pharmacokinetic studies to better adjust supplement doses [ 185 ].
Alpha-lipoic acid [ 186 ] and omega-3 fatty acids are two supplements that improve lipid and insulin sensitivity in women through their anti-inflammatory and antioxidant properties, although omega-3 fatty acids are high in calories [ 187 ]. Studies suggest that coenzyme Q10 has beneficial effects on glucose and lipid metabolism, insulin, HOMA-IR, and total testosterone levels in women with PCOS and can also improve ovarian function [ 188 , 189 , 190 ]. Vitamin E combined with coenzyme Q10 can improve IR and serum SHBG levels in PCOS [ 191 , 192 ]. Supplementation with probiotics, prebiotics, and synbiotics in women with PCOS can improve IR, protect the intestinal barrier, and regulate the immune system, lipid profile, and other metabolic disorders [ 193 , 194 ]. Recent studies have also found that a high-fibre diet consisting of whole grains, traditional Chinese medicinal foods, and prebiotics combined with the α-glucosidase inhibitor acarbose improved reproductive endocrine disorders, HI, and IR in women with PCOS compared with a high-fibre diet alone [ 195 ]. Animal studies have shown that aloe gel extract can act as a potential metabolic regulator of PCOS by controlling glucose homoeostasis, improving insulin secretion, and enhancing insulin-mediated glucose uptake to reduce glucose tolerance [ 221 , 222 ]. Clinical trials have also shown that l -carnitine supplementation effectively improves PCOS-IR by improving fatty acid β-oxidation and carbohydrate metabolism [ 194 , 195 , 221 ]. A recent trial showed that metformin combined with pioglitazone and acetylcarnitine improved IR and ovulation in women with PCOS more than metformin plus pioglitazone [ 199 ].
Traditional Chinese medicine
Traditional Chinese medicine (TCM) is often an important complement to modern Western medicine. Compounds isolated from Chinese herbs are particularly beneficial in improving metabolic disorders. Berberine (BBR) is an alkaloid that can relieve IR and treat PCOS by acting on a variety of insulin signalling pathways, including PPAR, MAPK, and AMPK. In recent years, it has been regarded as a safe and effective insulin sensitizer, although clinical data are lacking. BBR in combination with metformin appears to improve insulin sensitivity more [ 200 , 201 , 202 ]. Plant polysaccharides have many different pharmacological effects, and Dendrobium officinale has been shown to be effective in alleviating IR in PCOS [ 203 ]. Astragalus polysaccharides may improve insulin sensitivity in PCOS model rats by upregulating serum adiponectin levels and it may play an important role in the treatment of IR [ 204 ]. In clinical trial studies, saffron had a significant protective effect on FBG, HOMA-IR, and inflammatory levels of women with PCOS [ 205 , 206 ]. Pharmacological studies have also found that hehuan yin tang and yijing tang, as key components of various TCM prescriptions, can regulate androgen and insulin levels and improve PCOS-IR symptoms through various pharmacological pathways [ 207 , 223 ].
Acupoint application was also effective in improving metabolism and IR in obese women with PCOS [ 205 , 224 ]. Acupuncture is an important component of TCM. Systematic evaluation and some studies have shown that electroacupuncture may increase systemic glucose uptake and improve insulin sensitivity by activating the PCOS sympathetic nervous system and part of the parasympathetic nervous system [ 208 , 209 , 210 , 211 ], but it may not be as effective as metformin in improving insulin sensitivity in women with PCOS [ 212 ]. More large-sample clinical trials are needed to explore TCM as a potential treatment option for PCOS.
In general, women with PCOS develop IR owing to abnormal insulin signalling and metabolic dysfunction in insulin-responsive tissues, with a high incidence of IR in PCOS and a significant negative impact on health. Here, we discuss the molecular mechanisms, diagnosis, and protocol of IR-based PCOS. The pathogenesis of IR in PCOS is not completely clear, and apparently includes genetic and epigenetic changes, deficiency of insulin signal transduction, hyperandrogenaemia, obesity, and inflammation. IR in different PCOS tissues can selectively affect metabolic or mitotic pathways in many tissues, including the ovaries. Therefore, effective prevention and treatment options should be evaluated to improve IR in PCOS patients. Lifestyle interventions and insulin sensitization therapy can be effective strategies for improving insulin sensitivity, while increasing ovulation and reducing androgen levels. Among all of the insulin sensitizers, metformin is the most widely used in PCOS. However, all mentioned drugs for PCOS are still off-label and further studies with larger sample sizes are needed to evaluate the efficacy of these new treatments and provide new insights into the molecular mechanisms of IR in PCOS.
Availability of data and materials
All data generated or analysed during this study are included in this published article.
Abbreviations
- Insulin resistance
- Polycystic ovary syndrome
Hyperinsulinaemia
Hyperandrogenism
Oligo-anovulation
Luteinizing hormone
Insulin receptor
Protein kinase B
Body mass index
Frequently sampled intravenous glucose tolerance
Homoeostasis model assessment of insulin resistance
Liver-specific insulin receptor knockout
Nonalcoholic fatty liver disease
Sex hormone-binding globulin
Glycogen synthase kinase 3
Mammalian target of rapamycin
Glucose transporter 4
Mitogen-activated protein kinase-extracellular signal-regulated kinase
Protein kinase C
D -chiral inositol
Zawadzki JK, Dunaif A. Diagnostic criteria for polycystic ovary syndrome: towards a rational approach. Boston. 1992;77–84.
The Rotterdam ESHRE/ASRM-sponsored PCOS consensus workshop groxup. Revised 2003 consensus on diagnostic criteria and long-term health risks related to polycystic ovary syndrome (PCOS). Hum Reprod (Oxford, England). 2004;19(1):41–7.
Article Google Scholar
Azziz R, Carmina E, Dewailly D, Diamanti-Kandarakis E, Escobar-Morreale H, Futterweit W, et al. Positions statement: criteria for defining polycystic ovary syndrome as a predominantly hyperandrogenic syndrome: an Androgen Excess Society guideline. J Clin Endocrinol Metab. 2006;91(11):4237–45.
Article CAS Google Scholar
Bozdag G, Mumusoglu S, Zengin D, Karabulut E, Yildiz B. The prevalence and phenotypic features of polycystic ovary syndrome: a systematic review and meta-analysis. Hum Reprod (Oxford, England). 2016;31(12):2841–55.
Dapas M, Lin F, Nadkarni G, Sisk R, Legro R, Urbanek M, et al. Distinct subtypes of polycystic ovary syndrome with novel genetic associations: an unsupervised, phenotypic clustering analysis. PLoS Med. 2020;17(6): e1003132.
Yilmaz B, Vellanki P, Ata B, Yildiz B. Diabetes mellitus and insulin resistance in mothers, fathers, sisters, and brothers of women with polycystic ovary syndrome: a systematic review and meta-analysis. Fertil Steril. 2018;110(3):523-33.e14.
Liu Y, Qin Y, Wu B, Peng H, Li M, Luo H, et al. DNA methylation in polycystic ovary syndrome: emerging evidence and challenges. Reprod Toxicol (Elmsford, NY). 2022;111:11–9.
Merlo E, Silva I, Cardoso R, Graceli J. The obesogen tributyltin induces features of polycystic ovary syndrome (PCOS): a review. J Toxicol Environ Health Part B. 2018;21(3):181–206.
Sulaiman M, Al-Farsi Y, Al-Khaduri M, Saleh J, Waly M. Polycystic ovarian syndrome is linked to increased oxidative stress in Omani women. Int J Women’s Health. 2018;10:763–71.
Armanini D, Boscaro M, Bordin L, Sabbadin C. Controversies in the pathogenesis, diagnosis and treatment of PCOS: focus on insulin resistance, inflammation, and hyperandrogenism. Int J Mol Sci. 2022;23(8):4110.
Malamouli M, Levinger I, McAinch A, Trewin A, Rodgers R, Moreno-Asso A. The mitochondrial profile in women with polycystic ovary syndrome: impact of exercise. J Mol Endocrinol. 2022;68(3):R11–23.
Liu Q, Xie Y, Qu L, Zhang M, Mo Z. Dyslipidemia involvement in the development of polycystic ovary syndrome. Taiwan J Obstet Gynecol. 2019;58(4):447–53.
Sadeghi H, Adeli I, Calina D, Docea A, Mousavi T, Daniali M, et al. Polycystic ovary syndrome: a comprehensive review of pathogenesis, management, and drug repurposing. Int J Mol Sci. 2022;23(2):583.
Cassar S, Misso M, Hopkins W, Shaw C, Teede H, Stepto N. Insulin resistance in polycystic ovary syndrome: a systematic review and meta-analysis of euglycaemic-hyperinsulinaemic clamp studies. Hum Reprod (Oxford, England). 2016;31(11):2619–31.
Flavia T, Enzo B, Paolo M. Insulin resistance in a large cohort of women with polycystic ovary syndrome: a comparison between euglycaemic-hyperinsulinaemic clamp and surrogate indexes. Hum Reprod. 2017;32(12):2515–21.
González F, Considine RV, Abdelhadi OA, Acton AJ. saturated fat ingestion promotes lipopolysaccharide- mediated inflammation and insulin resistance in polycystic ovary syndrome. J Clin Endocrinol Metab. 2019;104:934–46.
Zhu S, Zhang B, Jiang X, Li Z, Chen ZJ. Metabolic disturbances in non-obese women with polycystic ovary syndrome: a systematic review and meta-analysis. Fertil Steril. 2019;111(1):168–77.
Flavia T, Michela V, Matteo M, Giulia F, Sabrina G, Tom F, et al. Insulin-mediated substrate use in women with different phenotypes of PCOS: the Role of Androgens. J Clin Endocrinol Metab. 2021;9:9.
Google Scholar
Hoeger KM, Dokras A, Piltonen T. Update on PCOS: Consequences, Challenges and Guiding Treatment. Journal of Clinical Endocrinology & Metabolism. 2020.
Moghetti P, Tosi F, Bonin C, Di Sarra D, Fiers T, Kaufman J-M, et al. Divergences in insulin resistance between the different phenotypes of the polycystic ovary syndrome. J Clin Endocrinol Metab. 2013;98(4):E628–37.
Bell GA, Sundaram R, Mumford SL, et al. Maternal polycystic ovarian syndrome and early offspring development. Hum Reprod. 2018;33(7):1307–15.
Chen Y, Guo J, Zhang Q, Zhang C. Insulin resistance is a risk factor for early miscarriage and macrosomia in patients with polycystic ovary syndrome from the first embryo transfer cycle: a retrospective cohort study. Front Endocrinol. 2022;13: 853473.
Hass RK, Dorte G, Mads N, Bo A, Marianne A. Development and risk factors of type 2 diabetes in a nationwide population of women with polycystic ovary syndrome. J Clin Endocrinol Metab. 2017;10:10.
Wekker V, van Dammen L, Koning A, Heida K, Painter R, Limpens J, et al. Long-term cardiometabolic disease risk in women with PCOS: a systematic review and meta-analysis. Hum Reprod Update. 2020;26(6):942–60.
Greenwood E, Pasch L, Cedars M, Legro R, Eisenberg E, Huddleston H. Insulin resistance is associated with depression risk in polycystic ovary syndrome. Fertil Steril. 2018;110(1):27–34.
Lee E, Oh B, Lee J, Kimm K, Lee S, Baek K. A novel single nucleotide polymorphism of INSR gene for polycystic ovary syndrome. Fertil Steril. 2008;89(5):1213–20.
Colilla S, Cox NJ, Ehrmann DA. Heritability of insulin secretion and insulin action in women with polycystic ovary syndrome and their first degree relatives 1. J Clin Endocrinol Metab. 2001;86(5):2027–31.
CAS Google Scholar
Franks S, Webber L, Goh M, Valentine A, White D, Conway G, et al. Ovarian morphology is a marker of heritable biochemical traits in sisters with polycystic ovaries. J Clin Endocrinol Metab. 2008;93(9):3396–402.
Sir-Petermann T, Codner E, Pérez V, Echiburú B, Maliqueo M, Ladrón de Guevara A, et al. Metabolic and reproductive features before and during puberty in daughters of women with polycystic ovary syndrome. J Clin Endocr. 2009;94(6):1923–30.
Xu Y, Qiao J. Association of insulin resistance and elevated androgen levels with Polycystic Ovarian Syndrome (PCOS): a review of literature. J Healthc Eng. 2022;2022:9240569.
McAllister J, Legro R, Modi B, Strauss J. Functional genomics of PCOS: from GWAS to molecular mechanisms. Trends Endocrinol Metab. 2015;26(3):118–24.
Jones M, Goodarzi M. Genetic determinants of polycystic ovary syndrome: progress and future directions. Fertil Steril. 2016;106(1):25–32.
Shi Y, Zhao H, Shi Y, Cao Y, Yang D, Li Z, et al. Genome-wide association study identifies eight new risk loci for polycystic ovary syndrome. Nat Genet. 2012;44(9):1020–5.
Dakshinamoorthy J, Jain P, Ramamoorthy T, Ayyappan R, Balasundaram U. Association of GWAS identified INSR variants (rs2059807 & rs1799817) with polycystic ovarian syndrome in Indian women. Int J Biol Macromol. 2020;144:663–70.
Mukherjee S, Shaikh N, Khavale S, Shinde G, Meherji P, Shah N, et al. Genetic variation in exon 17 of INSR is associated with insulin resistance and hyperandrogenemia among lean Indian women with polycystic ovary syndrome. Eur J Endocrinol. 2009;160(5):855–62.
Ioannidis A, Ikonomi E, Dimou N, Douma L, Bagos P. Polymorphisms of the insulin receptor and the insulin receptor substrates genes in polycystic ovary syndrome: a Mendelian randomization meta-analysis. Mol Genet Metab. 2010;99(2):174–83.
Ruan Y, Ma J, Xie X. Association of IRS-1 and IRS-2 genes polymorphisms with polycystic ovary syndrome: a meta-analysis. Endocr J. 2012;59(7):601–9.
Roldán B, San Millán J, Escobar-Morreale H. Genetic basis of metabolic abnormalities in polycystic ovary syndrome: implications for therapy. Am J Pharmacogenomics. 2004;4(2):93–107.
Hu M, Zhang Y, Guo X, Jia W, Liu G, Zhang J, et al. Hyperandrogenism and insulin resistance induce gravid uterine defects in association with mitochondrial dysfunction and aberrant ROS production. Am J Physiol Endocrinol Metab. 2019;316(5):E794–809.
Abbott D, Bacha F. Ontogeny of polycystic ovary syndrome and insulin resistance in utero and early childhood. Fertil Steril. 2013;100(1):2–11.
Jones R, Ozanne S. Fetal programming of glucose–insulin metabolism. Mol Cell Endocrinol. 2009;297(1):4–9.
Pandolfi C, Zugaro A, Lattanzio F, Necozione S, Barbonetti A, Colangeli M, et al. Low birth weight and later development of insulin resistance and biochemical/clinical features of polycystic ovary syndrome. Metabolism. 2008;57(7):999–1004.
Melo A, Vieira C, Barbieri M, Rosa-E-Silva A, Silva A, Cardoso V, et al. High prevalence of polycystic ovary syndrome in women born small for gestational age. Hum Reprod (Oxford, England). 2010;25(8):2124–31.
Saltiel A, Kahn C. Insulin signalling and the regulation of glucose and lipid metabolism. Nature. 2001;414(6865):799–806.
Lee JS, Pilch PF. The insulin receptor: Structure, function, and signaling. Am J Physiol. 1994;266(2 Pt 1):C319–34.
Choi K, Kim Y. Molecular mechanism of insulin resistance in obesity and type 2 diabetes. Korean J Intern Med. 2010;25(2):119–29.
Xu J, Dun J, Yang J, Zhang J, Lin Q, Huang M, et al. Letrozole rat model mimics human polycystic ovarian syndrome and changes in insulin signal pathways. Med Sci Monit. 2020;26:e923073.
Zeng X, Xie YJ, Liu YT, Long SL, Mo ZC. Polycystic ovarian syndrome: correlation between hyperandrogenism, insulin resistance and obesity. Clin Chim Acta. 2019;502:214–21.
Cantley LC, Neel BG. New insights into tumor suppression: PTEN suppresses tumor formation by restraining the phosphoinositide 3-kinase/AKT pathway. Proc Natl Acad Sci. 1999;96(8):4240–5.
Shaaban Z, Khoradmehr A, Amiri-Yekta A, Nowzari F, JafarzadehShirazi M, Tamadon A. Pathophysiologic mechanisms of insulin secretion and signaling-related genes in etiology of polycystic ovary syndrome. Genetics research. 2021;2021:7781823.
Evanthia DK, Andrea D. Insulin resistance and the polycystic ovary syndrome revisited: an update on mechanisms and implications. Endocr Rev. 2012;6:981–1030.
Nestler JE, Jakubowicz DJ, Aida FDV, Brik C, Quintero N, Medina F. Insulin stimulates testosterone biosynthesis by human thecal cells from women with polycystic ovary syndrome by activating its own receptor and using inositolglycan mediators as the signal transduction system. J Clin Endocrinol Metab. 1998;83(6):2001–5.
Samuel Varman T, Shulman GI. Mechanisms for insulin resistance: common threads and missing links. Cell. 2012;148:852–71.
Zhu S, Li Z, Hu C, Sun F, Wang C, Yuan H, et al. Imaging-based body fat distribution in polycystic ovary syndrome: a systematic review and meta-analysis. Front Endocrinol. 2021;12: 697223.
Kokosar M, Benrick A, Perfilyev A, Nilsson E, Kllman T, Ohlsson C, et al. A single bout of electroacupuncture remodels epigenetic and transcriptional changes in adipose tissue in polycystic ovary syndrome. Sci Rep. 2018;8(1):1878.
Polak A, Adamska A, Krentowska A, Łebkowska A, Hryniewicka J, Adamski M, et al. Body composition, serum concentrations of androgens and insulin resistance in different polycystic ovary syndrome phenotypes. J Clin Med. 2020;9(3):732.
Pasquali R, Gambineri A. The Endocrine Impact of Obesity and Body Habitus in the Polycystic Ovary Syndrome. Humana Press. 2006.
Abel ED, Peroni O, Kim JK, Kim Y-B, Boss O, Hadro E, et al. Adipose-selective targeting of the GLUT4 gene impairs insulin action in muscle and liver. Nature. 2001;409(6821):729–33.
Corbould A. Chronic testosterone treatment induces selective insulin resistance in subcutaneous adipocytes of women. J Endocrinol. 2007;192(3):585–94.
Perello M, Castrogiovanni D, Giovambattista A, Gaillard R, Spinedi E. Impairment in insulin sensitivity after early androgenization in the post-pubertal female rat. Life Sci. 2007;80(19):1792–8.
Puttabyatappa M, Lu C, Martin J, Chazenbalk G, Dumesic D, Padmanabhan V. Developmental programming: impact of prenatal testosterone excess on steroidal machinery and cell differentiation markers in visceral adipocytes of female sheep. Reprod Sci (Thousand Oaks, Calif). 2018;25(7):1010–23.
Dicker A, Rydén M, Näslund E, Muehlen I, Wirén M, Lafontan M, et al. Effect of testosterone on lipolysis in human pre-adipocytes from different fat depots. Diabetologia. 2004;47(3):420–8.
Paulukinas RD, Mesaros CA, Penning TM. Conversion of classical and 11-oxygenated androgens by Insulin-Induced AKR1C3 in a model of human PCOS Adipocytes. Endocrinology. 2022;7:7.
O’Reilly M, Kempegowda P, Walsh M, Taylor A, Manolopoulos K, Allwood J, et al. AKR1C3-mediated adipose androgen generation drives lipotoxicity in women with polycystic ovary syndrome. J Clin Endocrinol Metab. 2017;102(9):3327–39.
Brochu-Gaudreau K, Rehfeldt C, Blouin R, Bordignon V, Murphy B, Palin M. Adiponectin action from head to toe. Endocrine. 2010;37(1):11–32.
Nawrocki AR, Rajala MW, Tomas E, Pajvani UB, Saha AK, Trumbauer ME, et al. Mice lacking adiponectin show decreased hepatic insulin sensitivity and reduced responsiveness to peroxisome proliferator-activated receptor γ Agonists. J Biol Chem. 2006;281(5):2654–60.
Kershaw E, Flier J. Adipose tissue as an endocrine organ. J Clin Endocrinol Metab. 2004;89(6):2548–56.
Haiming C. Adipocytokines in obesity and metabolic disease. J Endocrinol. 2014;220(2):T47–59.
Zafari Zangeneh F, Naghizadeh M, Masoumi M. Polycystic ovary syndrome and circulating inflammatory markers. Int J Reprod Med. 2017;15(6):375–82.
DeFronzo R. Lilly lecture 1987. The triumvirate: beta-cell, muscle, liver. A collusion responsible for NIDDM. Diabetes. 1988;37(6):667–87.
Dunaif A, Wu X, Lee A, Diamanti-Kandarakis E. Defects in insulin receptor signaling in vivo in the polycystic ovary syndrome (PCOS). Am J Physiol Endocrinol Metab. 2001;281(2):E392–9.
Nilsson E, Benrick A, Kokosar M, Krook A, Lindgren E, Källman T, et al. Transcriptional and epigenetic changes influencing skeletal muscle metabolism in women with polycystic ovary syndrome. J Clin Endocrinol Metab. 2018;103(12):4465–77.
Corbould A, Kim Y, Youngren J, Pender C, Kahn B, Lee A, et al. Insulin resistance in the skeletal muscle of women with PCOS involves intrinsic and acquired defects in insulin signaling. Am J Physiol Endocrinol Metab. 2005;288(5):E1047–54.
Corbould A, Zhao H, Mirzoeva S, Aird F, Dunaif A. Enhanced mitogenic signaling in skeletal muscle of women with polycystic ovary syndrome. Diabetes. 2006;55(3):751–9.
Højlund K, Glintborg D, Andersen N, Birk J, Treebak J, Frøsig C, et al. Impaired insulin-stimulated phosphorylation of Akt and AS160 in skeletal muscle of women with polycystic ovary syndrome is reversed by pioglitazone treatment. Diabetes. 2008;57(2):357–66.
Hansen S, Svendsen P, Jeppesen J, Hoeg L, Andersen N, Kristensen J, et al. Molecular mechanisms in skeletal muscle underlying insulin resistance in women who are lean with polycystic ovary syndrome. J Clin Endocrinol Metab. 2019;104(5):1841–54.
Rajkhowa M, Brett S, Cuthbertson DJ, Lipina C, Ruiz-Alcaraz AJ, Thomas GE, Logie L, Petrie JR, Sutherland C. Insulin resistance in polycystic ovary syndrome is associated with defective regulation of ERK1/2 by insulin in skeletal muscle in vivo. Biochem J. 2009;418(3):665–71.
Allemand M, Irving B, Asmann Y, Klaus K, Tatpati L, Coddington C, et al. Effect of testosterone on insulin stimulated IRS1 Ser phosphorylation in primary rat myotubes–a potential model for PCOS-related insulin resistance. PLoS ONE. 2009;4(1): e4274.
Kazemi M, Pierson R, Parry S, Kaviani M, Chilibeck P. Obesity, but not hyperandrogenism or insulin resistance, predicts skeletal muscle mass in reproductive-aged women with polycystic ovary syndrome: A systematic review and meta-analysis of 45 observational studies. Obes Rev. 2021;22(8):e13255.
Hutchison SK, Teede HJ, Rachoń D, Harrison CL, Strauss BJ, Stepto NK. Effect of exercise training on insulin sensitivity, mitochondria and computed tomography muscle attenuation in overweight women with and without polycystic ovary syndrome. Diabetologia. 2012;55(5):1424–34.
Skov V, Glintborg D, Knudsen S, Jensen T, Kruse T, Tan Q, et al. Reduced expression of nuclear-encoded genes involved in mitochondrial oxidative metabolism in skeletal muscle of insulin-resistant women with polycystic ovary syndrome. Diabetes. 2007;56(9):2349–55.
Samuel V, Shulman G. The pathogenesis of insulin resistance: integrating signaling pathways and substrate flux. J Clin Investig. 2016;126(1):12–22.
Deswal R, Yadav A, Dang A. Sex hormone binding globulin - an important biomarker for predicting PCOS risk: a systematic review and meta-analysis. Syst Biol Reprod Med. 2018;64(1):12–24.
Michael M, Kulkarni R, Postic C, Previs S, Shulman G, Magnuson M, et al. Loss of insulin signaling in hepatocytes leads to severe insulin resistance and progressive hepatic dysfunction. Mol Cell. 2000;6(1):87–97.
Andrisse S, Feng M, Wang Z, Awe O, Yu L, Zhang H, et al. Androgen-induced insulin resistance is ameliorated by deletion of hepatic androgen receptor in females. FASEB J. 2021;35(10):e21921.
Cui P, Hu W, Ma T, Hu M, Tong X, Zhang F, et al. Long-term androgen excess induces insulin resistance and non-alcoholic fatty liver disease in PCOS-like rats. J Steroid Biochem Mol Biol. 2021;208: 105829.
Spremović Rađenović S, Pupovac M, Andjić M, Bila J, Srećković S, Gudović A, et al. Prevalence, risk factors, and pathophysiology of nonalcoholic fatty liver disease (NAFLD) in women with Polycystic Ovary Syndrome (PCOS). Biomedicines. 2022;10(1):131.
Targher G, Solagna E, Tosi F, Castello R, Spiazzi G, Zoppini G, et al. Abnormal serum alanine aminotransferase levels are associated with impaired insulin sensitivity in young women with polycystic ovary syndrome. J Endocrinol Invest. 2009;32(8):695–700.
Vassilatou E, Lafoyianni S, Vryonidou A, Ioannidis D, Kosma L, Katsoulis K, et al. Increased androgen bioavailability is associated with non-alcoholic fatty liver disease in women with polycystic ovary syndrome. Human Reprod (Oxford, England). 2010;25(1):212–20.
Baranova A, Tran T, Birerdinc A, Younossi Z. Systematic review: association of polycystic ovary syndrome with metabolic syndrome and non-alcoholic fatty liver disease. Aliment Pharmacol Ther. 2011;33(7):801–14.
Jeanes Y, Reeves S. Metabolic consequences of obesity and insulin resistance in polycystic ovary syndrome: diagnostic and methodological challenges. Nutr Res Rev. 2017;30(1):97–105.
Li Y, Chen C, Ma Y, Xiao J, Luo G, Li Y, et al. Multi-system reproductive metabolic disorder: significance for the pathogenesis and therapy of polycystic ovary syndrome (PCOS). Life Sci. 2019;228:167–75.
Franks S, Gilling-Smith C, Watson H, Willis D. Insulin action in the normal and polycystic ovary. Endocrinol Metab Clin North Am. 1999;28(2):361–78.
Rosenfield RL, Ehrmann DA. The Pathogenesis of Polycystic Ovary Syndrome (PCOS). Endocrine Reviews. 2016;37(5):er.2015-1104.
Wang J, Wu D, Guo H, Li M. Hyperandrogenemia and insulin resistance: the chief culprit of polycystic ovary syndrome. Life Sci. 2019;236: 116940.
Sanchez-Garrido M, Tena-Sempere M. Metabolic dysfunction in polycystic ovary syndrome: pathogenic role of androgen excess and potential therapeutic strategies. Mol Metab. 2020;35:100937.
Zhang C, Hu J, Wang W, Sun Y, Sun K. HMGB1-induced aberrant autophagy contributes to insulin resistance in granulosa cells in PCOS. FASEB J. 2020;34(7):9563–74.
Rice S, Christoforidis N, Gadd C, Nikolaou D, Seyani L, Donaldson A, et al. Impaired insulin-dependent glucose metabolism in granulosa-lutein cells from anovulatory women with polycystic ovaries. Human Reprod (Oxford, England). 2005;20(2):373–81.
Palomba S, Piltonen T, Giudice L. Endometrial function in women with polycystic ovary syndrome: a comprehensive review. Hum Reprod Update. 2021;27(3):584–618.
Zhang Y, Hu M, Yang F, Zhang Y, Ma S, Zhang D, et al. Increased uterine androgen receptor protein abundance results in implantation and mitochondrial defects in pregnant rats with hyperandrogenism and insulin resistance. J Mol Med (Berl). 2021;99(10):1427–46.
Xue J, Zhang H, Liu W, Liu M, Shi M, Wen Z, et al. Metformin inhibits growth of eutopic stromal cells from adenomyotic endometrium via AMPK activation and subsequent inhibition of AKT phosphorylation: a possible role in the treatment of adenomyosis. Reproduction (Cambridge, England). 2013;146(4):397–406.
Kim H, DiVall S, Deneau R, Wolfe A. Insulin regulation of GnRH gene expression through MAP kinase signaling pathways. Mol Cell Endocrinol. 2005;242:42–9.
Brüning JC, Gautam D, Burks DJ, Gillette J, Schubert M, Orban PC, et al. Role of brain insulin receptor in control of body weight and reproduction. Science. 2000;289(5487):2122–5.
Burks D, Font de Mora J, Schubert M, Withers D, Myers M, Towery H, et al. IRS-2 pathways integrate female reproduction and energy homeostasis. Nature. 2000;407(6802):377–82.
Zheng S-H, Du D-F, Li X-L. Leptin levels in women with polycystic ovary syndrome: a systematic review and a meta-analysis. Reprod Sci. 2017;24(5):656–70.
Hill J, Elias C, Fukuda M, Williams K, Berglund E, Holland W, et al. Direct insulin and leptin action on pro-opiomelanocortin neurons is required for normal glucose homeostasis and fertility. Cell Metab. 2010;11(4):286–97.
Adashi E, Hsueh A, Yen S. Insulin enhancement of luteinizing hormone and follicle-stimulating hormone release by cultured pituitary cells. Endocrinology. 1981;108(4):1441–9.
Brothers K, Wu S, DiVall S, Messmer M, Kahn C, Miller R, et al. Rescue of obesity-induced infertility in female mice due to a pituitary-specific knockout of the insulin receptor. Cell Metab. 2010;12(3):295–305.
Romualdi D, Giuliani M, Draisci G, Costantini B, Cristello F, Lanzone A, et al. Pioglitazone reduces the adrenal androgen response to corticotropin-releasing factor without changes in ACTH release in hyperinsulinemic women with polycystic ovary syndrome. Fertil Steril. 2007;88(1):131–8.
Sar M, Lubahn D, French F, Wilson E. Immunohistochemical localization of the androgen receptor in rat and human tissues. Endocrinology. 1990;127(6):3180–6.
Navarro G, Allard C, Morford J, Xu W, Liu S, Molinas A, et al. Androgen excess in pancreatic β cells and neurons predisposes female mice to type 2 diabetes. JCI Insight. 2018;3(12):e98607.
Shen H, Qiu L, Zhang Z, Qin Y, Cao C, Di W. Genome-wide methylated DNA immunoprecipitation analysis of patients with polycystic ovary syndrome. PLoS ONE. 2013;8(5): e64801.
Ting W, Yanyan Q, Jian H, Keqin H, Duan M. The relationship between insulin resistance and CpG island methylation of LMNA gene in polycystic ovary syndrome. Cell Biochem Biophys. 2013;67(3):1041–7.
Ilie I, Georgescu C. Polycystic ovary syndrome-epigenetic mechanisms and aberrant MicroRNA. Adv Clin Chem. 2015;71:25–45.
Long W, Zhao C, Ji C, Ding H, Cui Y, Guo X, et al. Characterization of serum microRNAs profile of PCOS and identification of novel non-invasive biomarkers. Cell Physiol Biochem. 2014;33(5):1304–15.
He F, Li Y. Role of gut microbiota in the development of insulin resistance and the mechanism underlying polycystic ovary syndrome: a review. J Ovarian Res. 2020;13(1):73.
Muscogiuri G, Altieri B, de Angelis C, Palomba S, Pivonello R, Colao A, et al. Shedding new light on female fertility: the role of vitamin D. Rev Endocr Metab Disord. 2017;18(3):273–83.
Ciebiera M, Esfandyari S, Siblini H, Prince L, Elkafas H, Wojtyła C, et al. Nutrition in gynecological diseases: current perspectives. Nutrients. 2021;13(4):1178.
Li S, Zhai J, Chu W, Geng X, Chen Z, Du Y. Altered circadian clock as a novel therapeutic target for constant darkness-induced insulin resistance and hyperandrogenism of polycystic ovary syndrome. Transl Res. 2020;219:13–29.
Pandi-Perumal S, Trakht I, Srinivasan V, Spence D, Maestroni G, Zisapel N, et al. Physiological effects of melatonin: role of melatonin receptors and signal transduction pathways. Prog Neurobiol. 2008;85(3):335–53.
Li C, Shi Y, You L, Wang L, Chen Z. Melatonin receptor 1A gene polymorphism associated with polycystic ovary syndrome. Gynecol Obstet Invest. 2011;72(2):130–4.
Xu X, Kou L, Wang H, Bo C, Song X. Genetic polymorphisms of melatonin receptors 1A and 1B may result in disordered lipid metabolism in obese patients with polycystic ovary syndrome. Mol Med Rep. 2019;19(3):2220–30.
Yi S, Xu J, Shi H, Li W, Li Q, Sun Y. Association between melatonin receptor gene polymorphisms and polycystic ovarian syndrome: a systematic review and meta-analysis. Biosci Rep. 2020;40(6):BSR20200824.
Merhi Z, Kandaraki E, Diamanti-Kandarakis E. Implications and future perspectives of AGEs in PCOS pathophysiology. Trends Endocrinol Metab. 2019;30(3):150–62.
Fenichel P, Chevalier N, Brucker-Davis F. Bisphenol A: an endocrine and metabolic disruptor. Ann Endocrinol. 2013;74(3):211–20.
Yang S, Yang C, Pei R, Li C, Li X, Huang X, et al. Investigation on the association of occupational stress with risk of polycystic ovary syndrome and mediating effects of HOMA-IR. Gynecol Endocrinol. 2018;34(11):961–4.
Steegers-Theunissen R, Wiegel R, Jansen P, Laven J, Sinclair K. Polycystic ovary syndrome: a brain disorder characterized by eating problems originating during puberty and adolescence. Int J Mol Sci. 2020;21(21):8211.
DeFronzo R, Tobin J, Andres R. Glucose clamp technique: a method for quantifying insulin secretion and resistance. Am J Physiol. 1979;237(3):E214–23.
Bergman RN. Orchestration of glucose homeostasis: from a small acorn to the California oak. Diabetes. 2007;56(6):1489–501.
Amisi C, Ciccozzi M, Pozzilli P. Wrist circumference: a new marker for insulin resistance in African women with polycystic ovary syndrome. World J Diabetes. 2020;11(2):42–51.
Otten J, Ahrén B, Olsson T. Surrogate measures of insulin sensitivity vs the hyperinsulinaemic-euglycaemic clamp: a meta-analysis. Diabetologia. 2014;57(9):1781–8.
Xiang S, Hua F, Tang Y, Jiang X, Zhuang Q, Qian F. Relationship between serum lipoprotein ratios and insulin resistance in polycystic ovary syndrome. Int J Endocrinol. 2012;2012:173281.
Ghaffarzad A, Amani R, Mehrzad Sadaghiani M, Darabi M, Cheraghian B. Correlation of serum lipoprotein ratios with insulin resistance in infertile women with polycystic ovarian syndrome: a case control study. Int J Fertil Steril. 2016;10(1):29–35.
He H, Feng J, Zhang S, Wang Y, Li J, Gao J, et al. The Apolipoprotein B/A1 Ratio is associated with metabolic syndrome components, insulin resistance, androgen hormones, and liver enzymes in women with polycystic ovary syndrome. Front Endocrinol. 2021;12: 773781.
Chen F, Liao Y, Chen M, Yin H, Chen G, Huang Q, et al. Evaluation of the efficacy of sex hormone-binding globulin in insulin resistance assessment based on HOMA-IR in patients with PCOS. Reprod Sci (Thousand Oaks, Calif). 2021;28(9):2504–13.
Peng Z, Sun Y, Lv X, Zhang H, Liu C, Dai S. Interleukin-6 levels in women with polycystic ovary syndrome: a systematic review and meta-analysis. PLoS ONE. 2016;11(2): e0148531.
Adamska A, Łebkowska A, Krentowska A, Adamski M, Kowalska I. The association between serum ferritin concentration and visceral adiposity estimated by whole-body DXA scan in women with polycystic ovary syndrome. Front Endocrinol. 2020;10:873.
Rizk N, Sharif E. Leptin as well as free leptin receptor is associated with polycystic ovary syndrome in young women. Int J Endocrinol. 2015;2015:927805.
Polak K, Czyzyk A, Simoncini T, Meczekalski B. New markers of insulin resistance in polycystic ovary syndrome. J Endocrinol Invest. 2017;40(1):1–8.
Amisi C. Markers of insulin resistance in Polycystic ovary syndrome women: an update. World J Diabetes. 2022;13(3):129–49.
Traub M. Assessing and treating insulin resistance in women with polycystic ovarian syndrome. World J Diabetes. 2011;2(3):33–40.
Aly J, Decherney A. Lifestyle modifications in PCOS. Clin Obstet Gynecol. 2021;64(1):83–9.
Gu Y, Zhou G, Zhou F, Wu Q, Ma C, Zhang Y, et al. Life modifications and PCOS: old story but new tales. Front Endocrinol. 2022;13: 808898.
Afrin S, AlAshqar A, El Sabeh M, Miyashita-Ishiwata M, Reschke L, Brennan J, et al. Diet and nutrition in gynecological disorders: a focus on clinical studies. Nutrients. 2021;13(6):1747.
Hirschberg A, Naessén S, Stridsberg M, Byström B, Holtet J. Impaired cholecystokinin secretion and disturbed appetite regulation in women with polycystic ovary syndrome. Gynecol Endocrinol. 2004;19(2):79–87.
Shang Y, Zhou H, He R, Lu W. Dietary modification for reproductive health in women with polycystic ovary syndrome: a systematic review and meta-analysis. Front Endocrinol. 2021;12: 735954.
Mei S, Ding J, Wang K, Ni Z, Yu J. Mediterranean diet combined with a low-carbohydrate dietary pattern in the treatment of overweight polycystic ovary syndrome patients. Front Nutr. 2022;9: 876620.
Escobar-Morreale H. Polycystic ovary syndrome: definition, aetiology, diagnosis and treatment. Nat Rev Endocrinol. 2018;14(5):270–84.
Richards C, Meah V, James P, Rees D, Lord R. HIIT’ing or MISS’ing the optimal management of polycystic ovary syndrome: a systematic review and meta-analysis of high- versus moderate-intensity exercise prescription. Front Physiol. 2021;12: 715881.
Patten R, McIlvenna L, Levinger I, Garnham A, Shorakae S, Parker A, et al. High-intensity training elicits greater improvements in cardio-metabolic and reproductive outcomes than moderate-intensity training in women with polycystic ovary syndrome: a randomized clinical trial. Human Reprod (Oxford, England). 2022;37(5):1018–29.
Patten R, Boyle R, Moholdt T, Kiel I, Hopkins W, Harrison C, et al. Exercise interventions in polycystic ovary syndrome: a systematic review and meta-analysis. Front Physiol. 2020;11:606.
Fernandez R, Moore V, Van Ryswyk E, Varcoe T, Rodgers R, March W, et al. Sleep disturbances in women with polycystic ovary syndrome: prevalence, pathophysiology, impact and management strategies. Nat Sci Sleep. 2018;10:45–64.
Ibrahim Y, Alorabi M, Abdelzaher W, Toni N, Thabet K, Hegazy A, et al. Diacerein ameliorates letrozole-induced polycystic ovarian syndrome in rats. Biomed Pharmacother. 2022;149:112870.
Zhu D, Chen Y, Huang J, Deng H, Shen X, Lu D, et al. Effects of metformin on pregnancy outcome, metabolic profile, and sex hormone levels in women with polycystic ovary syndrome and their offspring: a systematic review and meta-analysis. Ann Transl Med. 2022;10(7):418.
Teede H, Misso M, Costello M, Dokras A, Laven J, Moran L, et al. Recommendations from the international evidence-based guideline for the assessment and management of polycystic ovary syndrome. Clin Endocrinol. 2018;89(3):251–68.
Li Y, Tan J, Wang Q, Duan C, Hu Y, Huang W. Comparing the individual effects of metformin and rosiglitazone and their combination in obese women with polycystic ovary syndrome: a randomized controlled trial. Fertil Steril. 2020;113(1):197–204.
Carvajal R, Rosas C, Kohan K, Gabler F, Vantman D, Romero C, et al. Metformin augments the levels of molecules that regulate the expression of the insulin-dependent glucose transporter GLUT4 in the endometria of hyperinsulinemic PCOS patients. Human Reprod (Oxford, England). 2013;28(8):2235–44.
Chen Z, Wei H, Zhao X, Xin X, Peng L, Ning Y, et al. Metformin treatment alleviates polycystic ovary syndrome by decreasing the expression of MMP-2 and MMP-9 via H19/miR-29b-3p and AKT/mTOR/autophagy signaling pathways. J Cell Physiol. 2019;234(11):19964–76.
Oróstica M, Astorga I, Plaza-Parrochia F, Poblete C, Carvajal R, García V, et al. Metformin treatment regulates the expression of molecules involved in adiponectin and insulin signaling pathways in endometria from women with obesity-associated insulin resistance and PCOS. Int J Mol Sci. 2022;23(7):3922.
Sharpe A, Morley L, Tang T, Norman R, Balen A. Metformin for ovulation induction (excluding gonadotrophins) in women with polycystic ovary syndrome. Cochrane Database Syst Rev. 2019;12:CD013505.
Piątkowska-Chmiel I, Herbet M, Gawrońska-Grzywacz M, Dudka J. Regulation of neuroinflammatory signaling by PPARγ Agonist in mouse model of diabetes. Int J Mol Sci. 2022;23(10):5502.
Xu Y, Wu Y, Huang Q. Comparison of the effect between pioglitazone and metformin in treating patients with PCOS:a meta-analysis. Arch Gynecol Obstet. 2017;296(4):661–77.
Steiner CA, Janez A, Jensterle M, Reisinger K, Forst T, Pfützner A. Impact of treatment with rosiglitazone or metformin on biomarkers for insulin resistance and metabolic syndrome in patients with polycystic ovary syndrome. J Diabetes Sci Technol. 2007;1(2):211–7.
Salamun V, Jensterle M, Janez A, Vrtacnik BE. Liraglutide increases IVF pregnancy rates in obese PCOS women with poor response to first-line reproductive treatments: a pilot randomized study. Eur J Endocrinol. 2018;179(1):1–11.
Ge J, Wang D, Song W, Shen S, Ge W. The effectiveness and safety of liraglutide in treating overweight/obese patients with polycystic ovary syndrome: a meta-analysis. J Endocrinol Invest. 2022;45(2):261–73.
Bednarz K, Kowalczyk K, Cwynar M, Czapla D, Czarkowski W, Kmita D, et al. The Role of Glp-1 receptor agonists in insulin resistance with concomitant obesity treatment in polycystic ovary syndrome. Int J Mol Sci. 2022;23(8):4334.
Guo C, Huang T, Chen A, Chen X, Wang L, Shen F, et al. Glucagon-like peptide 1 improves insulin resistance in vitro through anti-inflammation of macrophages. Braz J Med Biol Res. 2016;49(12):e5826.
Knudsen L, Lau J. The discovery and development of liraglutide and semaglutide. Front Endocrinol. 2019;10:155.
Devin J, Nian H, Celedonio J, Wright P, Brown N. Sitagliptin decreases visceral fat and blood glucose in women with Polycystic Ovarian Syndrome. J Clin Endocrinol Metab. 2020;105(1):136–51.
Dardi I, Kouvatsos T, Jabbour S. SGLT2 inhibitors. Biochem Pharmacol. 2016;101:27–39.
Yaribeygi H, Sathyapalan T, Maleki M, Jamialahmadi T, Sahebkar A. Molecular mechanisms by which SGLT2 inhibitors can induce insulin sensitivity in diabetic milieu: a mechanistic review. Life Sci. 2020;240: 117090.
Tan S, Ignatenko S, Wagner F, Dokras A, Seufert J, Zwanziger D, et al. Licogliflozin versus placebo in women with polycystic ovary syndrome: a randomized, double-blind, phase 2 trial. Diabetes Obes Metab. 2021;23(11):2595–9.
Cai M, Shao X, Xing F, Zhang Y, Gao X, Zeng Q, et al. Efficacy of canagliflozin versus metformin in women with polycystic ovary syndrome: a randomized, open-label, noninferiority trial. Diabetes Obes Metab. 2022;24(2):312–20.
Graff S, Mario F, Ziegelmann P, Spritzer P. Effects of orlistat vs. metformin on weight loss-related clinical variables in women with PCOS: systematic review and meta-analysis. Int J Clin Pract. 2016;70(6):450–61.
Diamanti-Kandarakis E, Katsikis I, Piperi C, Alexandraki K, Panidis D. Effect of long-term orlistat treatment on serum levels of advanced glycation end-products in women with polycystic ovary syndrome. Clin Endocrinol. 2007;66(1):103–9.
Hutch C, Sandoval D. The Role of GLP-1 in the metabolic success of bariatric surgery. Endocrinology. 2017;158(12):4139–51.
Pareek M, Schauer PR, Kaplan LM, Leiter LA, Rubino F, Bhatt DL. Metabolic surgery: weight loss, diabetes, and beyond. J Am Coll Cardiol. 2018;71(6):670–87.
Daigle C, Brethauer S, Tu C, Petrick A, Morton J, Schauer P, et al. Which postoperative complications matter most after bariatric surgery? prioritizing quality improvement efforts to improve national outcomes. Surg Obes Relat Dis. 2018;14(5):652–7.
Switzer NJ, Marcil G, Prasad S, Debru E, Church N, Mitchell P, et al. Long-term hypovitaminosis D and secondary hyperparathyroidism outcomes of the Roux-en-Y gastric bypass: a systematic review. Obes Rev. 2017;18(5):560–6.
Simi G, Genazzani A, Obino M, Papini F, Pinelli S, Cela V, et al. Inositol and in vitro fertilization with embryo transfer. Int J Endocrinol. 2017;2017:5469409.
Genazzani A, Santagni S, Ricchieri F, Campedelli A, Rattighieri E, Chierchia E, et al. Myo-inositol modulates insulin and luteinizing hormone secretion in normal weight patients with polycystic ovary syndrome. J Obstet Gynaecol Res. 2014;40(5):1353–60.
Kamenov Z, Kolarov G, Gateva A, Carlomagno G, Genazzani A. Ovulation induction with myo-inositol alone and in combination with clomiphene citrate in polycystic ovarian syndrome patients with insulin resistance. Gynecol Endocrinol. 2015;31(2):131–5.
Gambioli R, Forte G, Buzzaccarini G, Unfer V, Laganà A. Myo-Inositol as a key supporter of fertility and physiological gestation. Pharmaceuticals (Basel, Switzerland). 2021;14(6):504.
Dinicola S, Unfer V, Facchinetti F, Soulage C, Greene N, Bizzarri M, et al. Inositols: from established knowledge to novel approaches. Int J Mol Sci. 2021;22(19):10575.
Laganà A, Forte G, Bizzarri M, Kamenov Z, Bianco B, Kaya C, et al. Inositols in the ovaries: activities and potential therapeutic applications. Expert Opin Drug Metab Toxicol. 2022;18(2):123–33.
Laganà A, Monti N, Fedeli V, Gullo G, Bizzarri M. Does Alpha-lipoic acid improve effects on polycystic ovary syndrome? Eur Rev Med Pharmacol Sci. 2022;26(4):1241–7.
Iervolino M, Lepore E, Forte G, Laganà A, Buzzaccarini G, Unfer V. Natural molecules in the management of Polycystic Ovary Syndrome (PCOS): an analytical review. Nutrients. 2021;13(5):1677.
Özcan P, Fıçıcıoğlu C, Kizilkale O, Yesiladali M, Tok O, Ozkan F, et al. Can Coenzyme Q10 supplementation protect the ovarian reserve against oxidative damage? J Assist Reprod Genet. 2016;33(9):1223–30.
Rahmani E, Jamilian M, Samimi M, Zarezade Mehrizi M, Aghadavod E, Akbari E, et al. The effects of coenzyme Q10 supplementation on gene expression related to insulin, lipid and inflammation in patients with polycystic ovary syndrome. Gynecol Endocrinol. 2018;34(3):217–22.
Samimi M, Zarezade Mehrizi M, Foroozanfard F, Akbari H, Jamilian M, Ahmadi S, et al. The effects of coenzyme Q10 supplementation on glucose metabolism and lipid profiles in women with polycystic ovary syndrome: a randomized, double-blind, placebo-controlled trial. Clin Endocrinol. 2017;86(4):560–6.
Tefagh G, Payab M, Qorbani M, Sharifi F, Sharifi Y, Ebrahimnegad Shirvani M, et al. Effect of vitamin E supplementation on cardiometabolic risk factors, inflammatory and oxidative markers and hormonal functions in PCOS (polycystic ovary syndrome): a systematic review and meta-analysis. Sci Rep. 2022;12(1):5770.
Izadi A, Ebrahimi S, Shirazi S, Taghizadeh S, Parizad M, Farzadi L, et al. Hormonal and metabolic effects of Coenzyme Q10 and/or Vitamin E in patients with polycystic ovary syndrome. J Clin Endocrinol Metab. 2019;104(2):319–27.
Ahmadi S, Jamilian M, Karamali M, Tajabadi-Ebrahimi M, Jafari P, Taghizadeh M, et al. Probiotic supplementation and the effects on weight loss, glycaemia and lipid profiles in women with polycystic ovary syndrome: a randomized, double-blind, placebo-controlled trial. Hum Fertil (Camb). 2017;20(4):254–61.
Li Y, Tan Y, Xia G, Shuai J. Effects of probiotics, prebiotics, and synbiotics on polycystic ovary syndrome: a systematic review and meta-analysis. Crit Rev Food Sci Nutr. 2021:1–17.
Wang X, Xu T, Liu R, Wu G, Gu L, Zhang Y, et al. High-fiber diet or combined with acarbose alleviates heterogeneous phenotypes of polycystic ovary syndrome by regulating gut microbiota. Front Endocrinol. 2021;12: 806331.
Samimi M, Jamilian M, Ebrahimi F, Rahimi M, Tajbakhsh B, Asemi Z. Oral carnitine supplementation reduces body weight and insulin resistance in women with polycystic ovary syndrome: a randomized, double-blind, placebo-controlled trial. Clin Endocrinol. 2016;84(6):851–7.
Petrillo T, Battipaglia C, Virmani M, Genazzani A, Genazzani A. Neuroendocrine effects of carnitines on reproductive impairments. Int J Mol Sci. 2021;22(19):10781.
Sangouni A, Pakravanfar F, Ghadiri-Anari A, Nadjarzadeh A, Fallahzadeh H, Hosseinzadeh M. The effect of L-carnitine supplementation on insulin resistance, sex hormone-binding globulin and lipid profile in overweight/obese women with polycystic ovary syndrome: a randomized clinical trial. Eur J Nutr. 2022;61(3):1199–207.
Tauqir S, Israr M, Rauf B, Malik MO, Shah M. Acetyl-L-Carnitine Ameliorates metabolic and endocrine alterations in women with PCOS: a double-blind randomized clinical trial. Adv Ther. 2021(9).
Wang Y, Fu X, Xu J, Wang Q, Kuang H. Systems pharmacology to investigate the interaction of berberine and other drugs in treating polycystic ovary syndrome. Sci Rep. 2016;6:28089.
Saleem F, Rizvi S. New therapeutic approaches in obesity and metabolic syndrome associated with polycystic ovary syndrome. Cureus. 2017;9(11): e1844.
Rondanelli M, Riva A, Petrangolini G, Allegrini P, Giacosa A, Fazia T, et al. Berberine phospholipid is an effective insulin sensitizer and improves metabolic and hormonal disorders in women with Polycystic Ovary Syndrome: a one-group pretest-post-test explanatory study. Nutrients. 2021;13(10):3665.
Chen Y, Song D, Jin WU, Jing Z, Liu L, Jiang R, et al. Improvement effects of Dendrobium candidum on insulin resistance in MKR mice and MIN6 cells. Journal of Jilin University(Medicine Edition). 2018.
Wei XJ, Chen XH, Weng XG, Wang T, Wang L. Effects of Astragalus Polysaccharides on the Insulin Resistance in Rats. Chinese Journal of Experimental Traditional Medical Formulae. 2011.
Rahmani J, Manzari N, Thompson J, Clark C, Villanueva G, Varkaneh H, et al. The effect of saffron on weight and lipid profile: a systematic review, meta-analysis, and dose-response of randomized clinical trials. Phytother Res. 2019;33(9):2244–55.
Rahimi G, Shams S, Aslani M. Effects of crocin supplementation on inflammatory markers, lipid profiles, insulin and cardioprotective indices in women with PCOS: a randomized, double-blind, placebo-controlled trial. Phytotherapy research : PTR. 2022;36(6):2605–15.
Wu Y, Li S, Zhu H, Zhuang Z, Shao M, Chen F, et al. Network pharmacology integrated with experimental validation reveals the regulatory mechanism of action of Hehuan Yin decoction in polycystic ovary syndrome with insulin resistance. J Ethnopharmacol. 2022;289: 115057.
Raja-Khan N, Stener-Victorin E, Wu X, Legro R. The physiological basis of complementary and alternative medicines for polycystic ovary syndrome. Am J Physiol Endocrinol Metab. 2011;301(1):E1–10.
Jia L, Feng J, Li J, Liu F, Xie L, Luo S, et al. The complementary and alternative medicine for polycystic ovary syndrome: a review of clinical application and mechanism. Evid Based Complement Alternat Med. 2021;2021:5555315.
Benrick A, Kokosar M, Hu M, Larsson M, Maliqueo M, Marcondes R, et al. Autonomic nervous system activation mediates the increase in whole-body glucose uptake in response to electroacupuncture. FASEB J. 2017;31(8):3288–97.
Zheng R, Qing P, Han M, Song J, Hu M, Ma H, et al. The effect of acupuncture on glucose metabolism and lipid profiles in patients with PCOS: a systematic review and meta-analysis of randomized controlled trials. Evid Based Complement Alternat Med. 2021;2021:5555028.
Wen Q, Hu M, Lai M, Li J, Hu Z, Quan K, et al. Effect of acupuncture and metformin on insulin sensitivity in women with polycystic ovary syndrome and insulin resistance: a three-armed randomized controlled trial. Human Reprod (Oxford, England). 2022;37(3):542–52.
Glueck C, Goldenberg N. Characteristics of obesity in polycystic ovary syndrome: Etiology, treatment, and genetics. Metabolism. 2019;92:108–20.
Xing C, Li C, He B. Insulin sensitizers for improving the endocrine and metabolic profile in overweight women with PCOS. J Clin Endocrinol Metab. 2020;105(9):2950–63.
Zhao H, Xing C, Zhang J, He B. Comparative efficacy of oral insulin sensitizers metformin, thiazolidinediones, inositol, and berberine in improving endocrine and metabolic profiles in women with PCOS: a network meta-analysis. Reprod Health. 2021;18(1):171.
Müller TD, Finan B, Bloom SR, D’Alessio D, Drucker DJ, Flatt PR, et al. Glucagon-like peptide 1 (GLP-1). Molecular Metabolism. 2019;30:72–130.
Lee Y-S, Park M-S, Choung J-S, Kim S-S, Oh H-H, Choi C-S, et al. Glucagon-like peptide-1 inhibits adipose tissue macrophage infiltration and inflammation in an obese mouse model of diabetes. Diabetologia. 2012;55(9):2456–68.
Siamashvili M, Davis S. Update on the effects of GLP-1 receptor agonists for the treatment of polycystic ovary syndrome. Expert Rev Clin Pharmacol. 2021;14(9):1081–9.
DeFronzo R, Norton L, Abdul-Ghani M. Renal, metabolic and cardiovascular considerations of SGLT2 inhibition. Nat Rev Nephrol. 2017;13(1):11–26.
Marinkovic-Radosevic J, Cigrovski Berkovic M, Kruezi E, Bilic-Curcic I, Mrzljak A. Exploring new treatment options for polycystic ovary syndrome: review of a novel antidiabetic agent SGLT2 inhibitor. World J Diabetes. 2021;12(7):932–8.
Nampoothiri L, Desai B, Maharjan R. Aloe barbadensis Mill. formulation restores lipid profile to normal in a letrozole-induced polycystic ovarian syndrome rat model. Pharmacognosy Res. 2012;4(2):109–15.
Dey A, Dhadhal S, Maharjan R, Nagar PS, Nampoothiri L. Partially purified non-polar phytocomponents from Aloe barbadensis Mill. gel restores metabolic and reproductive comorbidities in letrozole-induced polycystic ovary syndrome rodent model- an “in-vivo” study. Journal of Ethnopharmacology. 2022;291:115161.
Lin Y, Xiang L, Li X, Tang Q, Meng F, Chen W. Exploring the Mechanism of Yi-Jing Decoction in Treating Polycystic Ovary Syndrome by Using Network Pharmacology. Curr Med Chem. 2022.
Shao C, Dong W, Zhang H. Application of Guijiaosan Shenque acupoint paste can improve the scores of obesity, endocrine and TCM symptoms in treating obese polycystic ovary syndrome. Am J Transl Res. 2021;13(9):10694–702.
Download references
Acknowledgements
This work was supported by a grant from the National Natural Science Foundation of China (Grant no. 81570765), the Joint Program of Liaoning Provincial Department of Science and Technology on Livelihood science and Technology, and the 345 Talent Project of ShengJing Hospital of China Medical University.
Author information
Authors and affiliations.
Department of Endocrinology, Shengjing Hospital, China Medical University, Shenyang, Liaoning, 110000, People’s Republic of China
Han Zhao, Jiaqi Zhang, Xiangyi Cheng, Xiaozhao Nie & Bing He
You can also search for this author in PubMed Google Scholar
Contributions
BH and HZ conceived the idea; HZ reviewed the literature; HZ designed the study and wrote the manuscript and designed the figures and tables; BH, JZ, XC, and XN critically revised the manuscript. All authors participated in the revision the manuscript. All authors participated in the discussion of the analysis and interpretation of data in this article. The author(s) read and approved the final manuscript.
Corresponding author
Correspondence to Bing He .
Ethics declarations
Ethics approval and consent to participate.
Not applicable.
Consent for publication
Competing interests.
The authors have no conflicts of interest to declare.
Additional information
Publisher’s note.
Springer Nature remains neutral with regard to jurisdictional claims in published maps and institutional affiliations.
Rights and permissions
Open Access This article is licensed under a Creative Commons Attribution 4.0 International License, which permits use, sharing, adaptation, distribution and reproduction in any medium or format, as long as you give appropriate credit to the original author(s) and the source, provide a link to the Creative Commons licence, and indicate if changes were made. The images or other third party material in this article are included in the article's Creative Commons licence, unless indicated otherwise in a credit line to the material. If material is not included in the article's Creative Commons licence and your intended use is not permitted by statutory regulation or exceeds the permitted use, you will need to obtain permission directly from the copyright holder. To view a copy of this licence, visit http://creativecommons.org/licenses/by/4.0/ . The Creative Commons Public Domain Dedication waiver ( http://creativecommons.org/publicdomain/zero/1.0/ ) applies to the data made available in this article, unless otherwise stated in a credit line to the data.
Reprints and permissions
About this article
Cite this article.
Zhao, H., Zhang, J., Cheng, X. et al. Insulin resistance in polycystic ovary syndrome across various tissues: an updated review of pathogenesis, evaluation, and treatment. J Ovarian Res 16 , 9 (2023). https://doi.org/10.1186/s13048-022-01091-0
Download citation
Received : 07 July 2022
Accepted : 26 December 2022
Published : 11 January 2023
DOI : https://doi.org/10.1186/s13048-022-01091-0
Share this article
Anyone you share the following link with will be able to read this content:
Sorry, a shareable link is not currently available for this article.
Provided by the Springer Nature SharedIt content-sharing initiative
- Hyperandrogenaemia
Journal of Ovarian Research
ISSN: 1757-2215
- General enquiries: [email protected]
- Search Menu
- Volume 8, Issue 6, June 2024 (In Progress)
- Volume 8, Issue 5, May 2024
- Diabetes, Pancreatic and Gastrointestinal Hormones
- Lipids and Cardiovascular
- Obesity and Adipocyte Biology
- Parathyroid, Bone, and Mineral Metabolism
- Pituitary and Neuroendocrinology
- Advance Articles
- Expert Endocrine Consult Articles
- ENDO Meeting Abstracts
- Obesity Research
- Thematic Issues
- Clinical Practice Guidelines
- Endocrine Reviews
- Endocrinology
- Journal of the Endocrine Society
- The Journal of Clinical Endocrinology & Metabolism
- JCEM Case Reports
- Molecular Endocrinology
- Endocrine Society Journals
- Author Guidelines
- Submission Site
- Open Access
- Why Publish with the Endocrine Society?
- Advertising & Corporate Services
- Reprints, ePrints, Supplements
- About Journal of the Endocrine Society
- Editorial Board
- Author Resources
- Reviewer Resources
- Rights & Permissions
- Other Society Publications
- Member Access
- Terms and Conditions
- Journals on Oxford Academic
- Books on Oxford Academic

Article Contents
1. pathophysiology, 2. diagnosis of pcos, 3. treatment of adolescent pcos, acknowledgments, additional information, references and notes.
- < Previous
Polycystic Ovary Syndrome: Pathophysiology, Presentation, and Treatment With Emphasis on Adolescent Girls

- Article contents
- Figures & tables
- Supplementary Data
Selma Feldman Witchel, Sharon E Oberfield, Alexia S Peña, Polycystic Ovary Syndrome: Pathophysiology, Presentation, and Treatment With Emphasis on Adolescent Girls, Journal of the Endocrine Society , Volume 3, Issue 8, August 2019, Pages 1545–1573, https://doi.org/10.1210/js.2019-00078
- Permissions Icon Permissions
Polycystic ovary syndrome (PCOS) is a heterogeneous disorder characterized by hyperandrogenism and chronic anovulation. Depending on diagnostic criteria, 6% to 20% of reproductive aged women are affected. Symptoms of PCOS arise during the early pubertal years. Both normal female pubertal development and PCOS are characterized by irregular menstrual cycles, anovulation, and acne. Owing to the complicated interwoven pathophysiology, discerning the inciting causes is challenging. Most available clinical data communicate findings and outcomes in adult women. Whereas the Rotterdam criteria are accepted for adult women, different diagnostic criteria for PCOS in adolescent girls have been delineated. Diagnostic features for adolescent girls are menstrual irregularity, clinical hyperandrogenism, and/or hyperandrogenemia. Pelvic ultrasound findings are not needed for the diagnosis of PCOS in adolescent girls. Even before definitive diagnosis of PCOS, adolescents with clinical signs of androgen excess and oligomenorrhea/amenorrhea, features of PCOS, can be regarded as being “at risk for PCOS.” Management of both those at risk for PCOS and those with a confirmed PCOS diagnosis includes education, healthy lifestyle interventions, and therapeutic interventions targeting their symptoms. Interventions can include metformin, combined oral contraceptive pills, spironolactone, and local treatments for hirsutism and acne. In addition to ascertaining for associated comorbidities, management should also include regular follow-up visits and planned transition to adult care providers. Comprehensive knowledge regarding the pathogenesis of PCOS will enable earlier identification of girls with high propensity to develop PCOS. Timely implementation of individualized therapeutic interventions will improve overall management of PCOS during adolescence, prevent associated comorbidities, and improve quality of life.
The female hypothalamic–pituitary–ovarian (HPO) axis is a meticulously synchronized and tightly regulated network ultimately responsible for reproductive competence and survival of the species. The HPO axis responds to internal signals ( i.e. , hormonal and neuronal) and external factors ( i.e. , environment influences). Beginning during gestation, these factors impact future generations through epigenetic factors affecting the brain and the developing germ cells [ 1 ].
Polycystic ovary syndrome (PCOS), a disorder primarily characterized by signs and symptoms of androgen excess and ovulatory dysfunction, disrupts HPO axis function. Depending on diagnostic criteria, this disorder affects ∼6% to 20% of reproductive aged women [ 2 , 3 ]. Typical clinical features include hirsutism, irregular menses, chronic anovulation, and infertility. The persistent hyperandrogenism is associated with impaired hypothalamic–pituitary feedback, LH hypersecretion, premature granulosa cell luteinization, aberrant oocyte maturation, and premature arrest of activated primary follicles [ 4 ].
By the time the diagnosis is established, PCOS presents as a phenotype reflecting a self-perpetuating vicious cycle involving neuroendocrine, metabolic, and ovarian dysfunction. Over the years, numerous hypotheses have been proposed regarding the proximate physiologic origins for PCOS. PCOS reflects the interactions among multiple proteins and genes influenced by epigenetic and environmental factors ( Fig. 1 ) [ 5 ]. Specific sections of this article deconstruct the factors contributing to the development of PCOS in humans and preclinical models. Clinical and biochemical hyperandrogenism are major features of PCOS.

Factors contributing to PCOS phenotype. PCOS encompasses a woman’s life cycle. Factors potentially impacting the pathophysiology of PCOS are shown in circles. Not all factors affect each individual. PCOS epitomizes a biologic network of interacting neuroendocrine, hormonal, metabolic, genetic, and environmental influences.
PCOS develops during the early pubertal years [ 6 ]. However, most relevant information has been accrued through clinical studies involving adult women in which referral bias focuses on investigation of the more severe phenotypes [ 7 ]. Preclinical models involving animal and in vitro studies supplement clinical investigation and benefit from other approaches to study this complex disorder. Recent clinical, experimental, and genetic data emphasize neuroendocrine involvement in the pathophysiology of PCOS.
A. Ovary, Adrenal, and Androgen Excess
PCOS is characterized by excessive ovarian and/or adrenal androgen secretion. Intrinsic ovarian factors such as altered steroidogenesis and factors external to the ovary such as hyperinsulinemia contribute to the excessive ovarian androgen production. Characteristic features include more growing follicles in women with PCOS compared with normal controls with premature growth arrest of antral follicles at 5 to 8 mm. The classic ovarian phenotype of enlarged ovaries with string-of-pearl morphology and theca interstitial hyperplasia reflects androgen exposure; this morphology has also been observed in women with congenital adrenal hyperplasia (CAH) and female-to-male transgender individuals [ 8 ]. Distorted interactions among the endocrine, paracrine, and autocrine factors responsible for follicular maturation may contribute to ovarian dysregulation in PCOS.
The stages of follicular maturation are briefly reviewed ( Fig. 2 ). Developing during gestation, primordial follicles are comprised of meiotically arrested oocytes surrounded by pregranulosa cells. Hence, a woman’s ovaries have been exposed to the ambient maternal environment during gestation. Ovaries are relatively quiescent until the onset of puberty. Detailed knowledge regarding follicular morphology in prepubertal and early pubertal ovaries is lacking. Ovarian tissue obtained from prepubertal and early pubertal girls shows differences in follicle morphology and growth potential. Specifically, prepubertal ovaries contain a high proportion of abnormal nongrowing follicles, which are not found in pubertal ovaries [ 9 ]. The physiologic relevance of this finding is unclear.

Ovarian follicle development. This illustration shows ovarian follicular development during developmental periods.
The precise signaling mechanisms initiating follicular activation are poorly understood. Presumably a balance of factors influences the options—continuation in a resting state or activation. One such factor appears to be follicle density [ 10 ]. Following activation from the resting pool, initial follicular growth is gonadotropin-independent until the antral stage.
Anti-Müllerian hormone (AMH), a glycoprotein secreted by granulosa cells, inhibits initial follicular recruitment and indicates follicular reserve. In contrast to mice where AMH inhibits preantral follicle growth and antral follicle maturation, AMH appears to promote growth of preantral follicles to the antral stage in nonhuman primate (NHP) ovaries [ 11 , 12 ]. Peak AMH concentrations are found in antral follicles. Once FSH-stimulated granulosa cell estradiol concentrations achieve the necessary threshold, estradiol suppresses AMH expression [ 13 ].
Despite prior assumptions that androgens negatively impact follicles, androgens synthesized in preantral follicle theca cells promote growth of preantral and antral follicles and induce granulosa cell FSH receptor (FSHR) expression in early antral follicles [ 14 ]. Androgens promote aromatase expression and, ultimately, LH/chorionic gonadotropin receptor (LHCGR) expression in granulosa cells. As a follicle matures, androgens appear to inhibit proliferation and promote apoptosis. This biphasic androgen action was initially demonstrated in an NHP, the marmoset; androgens augmented FSH action in small antral follicles but had an inhibitory effect in larger follicles [ 15 ].
Androgen actions are mediated by androgen receptors (ARs), which are expressed in theca cells, granulosa cells, oocytes, and stromal cells [ 16 ]. Both canonical androgen signaling where AR functions as ligand-dependent transcription factor and nongenomic signaling occur. Peak AR gene expression occurs in small antral follicles (∼6 mm in diameter) and decreases in antral and preovulatory follicles [ 17 ].
Typically, one follicle is “selected” as the dominant follicle [ 18 ]. With increasing estrogen secretion, pituitary FSH secretion declines due to negative feedback. The dominant follicle compensates for this loss of FSH stimulation through increased LHCGR expression and increased responsiveness to LH stimulation. Subordinate follicles undergo atresia, presumably due to relative FSH deficiency and androgen excess. Upon achieving a sufficient estradiol concentration, neuroendocrine mechanisms trigger the LH surge to induce ovulation.
Under normal circumstances, the ovarian stroma provides a structural framework undergoing dynamic changes to support follicular growth. However, the ovarian stroma from women with PCOS tends to be more rigid. The developing oocyte and its surrounding scaffolding rely on endocrine, paracrine, and autocrine signaling mechanisms to maintain cell-to-cell communication and assure synchronized developmental progression. Aberrant development during these earliest stages of follicular growth likely contributes to the ovarian aspects of PCOS [ 19 ]. Another feature of PCOS ovaries is accelerated transition from primordial to growing follicles with increased numbers of 2- to 3-mm and 3- to 4-mm follicles [ 20 , 21 ]. AMH concentrations correlate with the number of these small antral follicles [ 22 ]. The growing follicle is exposed to an atypical environment with increased LH, insulin, androgen, and AMH concentrations accompanied by insufficient FSH concentrations [ 19 ]. Additional differences in PCOS ovaries include factors impacting vascular function and immune responsiveness [ 23 ].
Additionally, intrinsic alterations in ovarian steroidogenesis likely contribute to excessive ovarian androgen production. Available data document constitutively increased androgen production and CYP17A1 expression in cultured theca cells isolated from PCOS ovaries [ 24–26 ]. Steroidogenesis in the ovary involves both theca and granulosa cells. The theca cells produce ovarian androgens, which are converted to estrogens in the granulosa cell due to the actions of FSH-stimulated aromatase.
One interesting locus identified through genetic studies is DENND1A (see “H. Genetics” below). Overexpression of the alternative spliced variant, DENND1A.V2 , of this gene recapitulated a PCOS phenotype in cultured theca cells obtained from normal women, indicating a role for this variant in the excessive theca cell androgen production [ 27 ]. Overexpression of DENND1A.V2 in an adrenal cell line led to increased expression of the mRNAs for CYP17A1 and CYP11A1 . However, the mechanisms responsible for increased expression of this alternatively spliced variant of DENND1A remain to be elucidated [ 28 ].
The adrenal zona reticularis is responsible for biosynthesis of the C-19 adrenal androgens, including dehydroepiandrosterone (DHEA), DHEA sulfate (DHEAS), androstenedione, and testosterone. At least three distinct adrenal pathways contribute to androgen synthesis: (i) canonical/classical, (ii) “alternative backdoor,” and (iii) 11-oxo-androgens ( Fig. 3 ). In the canonical/classical pathway, progesterone is successively transformed by the enzyme 17 α -hydroxylase/17,20-lyase (P450c17) to DHEA, which is subsequently converted by 3 β -hydroxysteroid dehydrogenase type 2 to androstenedione. The alternative backdoor pathway bypasses the usual steroid hormone intermediates, DHEA, androstenedione, and testosterone, to produce dihydrotestosterone [ 29 ]. This pathway likely contributes to virilization of the external genitalia among girls with classical CAH and normal male external genital development [ 30 , 31 ]. The extent of the contribution of the alternative backdoor pathway to adrenal and ovarian steroid biosynthesis in PCOS is unclear [ 32 ].

Androgen biosynthesis. This illustration shows the classical/canonical, alternative backdoor, and 11-oxo-steroid pathways for androgen biosynthesis.
The adrenal steroidogenic enzyme, 11 β -hydroxylase (P450c11B1), encoded by CYP11B1 , is expressed in both zona fasciculata and zona reticularis; this enzyme converts androstenedione and testosterone to their respective 11 β -hydroxyl derivatives, 11 β -hydroxy-androstenedione and 11 β -hydroxy-testosterone. Both 11 β -hydroxy-androstenedione and 11 β -hydroxy-testosterone can be converted to their 11-keto counterparts, 11-ketoandrostenedione and 11-ketotestosterone. Testosterone, DHT, 11-ketotestosterone, and 11-ketodihydrotestosterone bind to the human AR and promote AR-regulated gene expression [ 33 ]. The concentrations of these 11-oxygenated steroids were reported to be higher in women with PCOS than among healthy premenopausal women [ 34 ]. Urinary steroid profiling using 24-hour urine collections in a relatively small number of women with PCOS and controls found androstanediol concentrations to discriminate between PCOS and controls; the overall pattern of steroid hormone excretion indicated enhanced androgen biosynthesis via canonical/classical, alternative backdoor, and 11-oxygenated steroid pathways rather than a specific steroid enzyme disorder [ 35 ].
A-1. Preclinical models
One unresolved conundrum regarding folliculogenesis is the regulation and interrelationships between androgens and AMH. Androgens and AMH are essential for normal cyclic ovulation. Using short hairpin RNAs to decrease AMH expression in macaques, preantral follicle growth and survival were reduced. Cotreatment with supplemental AMH overcame these effects. These results emphasize the important role of AMH as a critical factor to promote preantral follicle survival and growth in primates [ 36 ]. AMH treatment of cultured antral stage rhesus macaque follicles decreased estradiol production compared with untreated follicles despite similar follicle size [ 12 ]. Hence, AMH appears to have a dual role: whereas AMH promotes preantral follicle survival, it negatively impacts later stages of antral follicle maturation [ 12 ].
In a series of in vivo and in vitro experiments, a subset of hypothalamic GnRH neurons, both mouse and human, were shown to express AMR receptors; AMH treatment increased GnRH-dependent LH pulsatility and secretion [ 37 ]. Using a mouse model, AMH treatment of pregnant mice was associated with diminished placental metabolism of testosterone to estradiol, decreased aromatase expression, masculinization of exposed female offspring, estrus cycle disturbances, increased LH pulse frequency, brain masculinization, and infertility compared with unexposed mice; postnatal GnRH antagonist treatment reversed this PCOS-like phenotype [ 38 ]. These data suggest that excessive prenatal AMH exposure could promote the aberrant neuroendocrine function typical of PCOS and that AMH can modulate GnRH neuron function [ 37 , 38 ].
Knockout mice have been used to explore consequences of gene deletions. Using a postnatal androgen PCOS model, global AR knockout mice were protected from DHT-induced PCOS-like features. Curiously, neuron-specific AR knockout mice were protected from DHT-induced ovarian dysfunction and several metabolic traits, reinforcing a role for extraovarian tissues in the pathophysiology of PCOS [ 39 ]. Hence, intricate interrelationships exist between androgens, AMH, follicle growth, metabolism, and neuroendocrine factors in PCOS.
Recently, a group of naturally hyperandrogenic female rhesus monkeys have been described. The high testosterone animals had increased LH, AMH, and androstenedione concentrations. Additionally, five of the six high-testosterone monkeys had no live offspring [ 40 ]. Future study of these animals will provide insight into the pathogenesis of PCOS.
B. Neuroendocrine Factors
Increased LH pulse frequency, LH pulse amplitude, and increased LH/FSH ratios are described in women with PCOS. The initial features of PCOS emerge during the early pubertal years, concomitant with reactivation of the hypothalamic GnRH pulse generator, increased gonadotropin secretion, and subsequent increased ovarian estrogen production. Loci identified in the genome-wide association studies (GWASs) studies include LHCGR , FSHR , and FSH- β polypeptide ( FSHB ) genes, emphasizing neuroendocrine contributions to PCOS pathophysiology (see H. Genetics below).
Hypothalamic neurons in the arcuate nucleus secrete kisspeptin, neurokinin B, and dynorphin. These neurons, labeled as the KNDy neurons, are the leading contenders for the hypothalamic GnRH pulse generator because of the colocalization of these three peptides and their roles in episodic GnRH secretion [ 41 ]. Rather than initiating puberty, the GnRH pulse generator and GnRH neurons represent downstream nodes modulated by other hormones and neurosecretory factors [ 42 ]. In other words, activation of excitatory inputs and inactivation of inhibitory inputs moderated by multiple influences regulate the output of the GnRH pulse generator to govern the timing of puberty [ 43–45 ]. This process culminates in increased GnRH and gonadotropin secretion.
The hypothalamic GnRH neurons secrete GnRH in discrete pulses that travel through the median eminence to the pituitary gonadotrophs, resulting in pulsatile LH and FSH secretion [ 46 ]. LH and FSH pulse frequencies are modulated by GnRH pulse frequency. Increased GnRH pulse frequency increases LH pulse frequency and decreases FSH pulse frequency [ 47 ]. The GnRH neurons integrate diverse influences, decode metabolic signals, and serve as the output “managers” of the HPO axis [ 48 , 49 ].
Increased LH pulse amplitude and pulse frequency observed in PCOS are likely driven by increased pulsatile GnRH secretion. Manipulation of the hypothalamic kisspeptin–neurokinin B–GnRH pathway with an NK3 receptor antagonist, AZD4901, reduced serum LH pulse frequency and, subsequently, serum LH and testosterone concentrations. These data suggest the possibility of targeting neuroendocrine pathophysiology to treat HPO axis dysfunction in PCOS [ 50 ].
GnRH neurons express estrogen receptor- β , but they do not express AR, progesterone receptor, or estrogen receptor- α . Hence, steroid-mediated negative feedback is indirect and is mediated through the hypothalamic neuronal network upstream of the GnRH neuron. This negative feedback mechanism is impaired in some women with PCOS who appear to require higher progesterone and estradiol concentrations. This effect can be abrogated with androgen antagonist treatment [ 51 ].
One conundrum is that LH hypersecretion is less obvious in women with obesity with PCOS. Although GnRH and LH pulses generally exhibit a 1:1 ratio, preclinical data exist suggesting that a faster GnRH pulse frequency may be associated with decreased LH secretion [ 52 ]. Potential explanations for this mismatch between GnRH and LH pulses include the longer half-life of LH obscuring pulse detection, exhaustion of the pituitary pool of readily releasable LH, or lower amplitude GnRH pulses [ 53 ]. Measurement of circulating kisspeptin and LH concentrations showed temporal kisspeptin–LH pulse coupling in eumenorrheic women with PCOS; however, a greater frequency of kisspeptin pulses was associated with a loss of temporal coupling in women with oligomenorrhea with PCOS [ 54 ]. This study identified dissociated coupling of kisspeptin and LH pulses in women with oligomenorrhea with PCOS.
Tanycytes are specialized nonciliated cells lining the floor of the third ventricle. These polarized cells contribute to regulation of reproduction and metabolism in the median eminence. Specifically, tanycytes affect GnRH secretion, generate active forms of thyroid hormone, and influence exchange of signaling factors such as leptin between the blood and hypothalamic extracellular fluid [ 55 ]. Dynamic structural remodeling of tanycytes modulates GnRH neuron access to the pituitary portal system. Leptin and ghrelin enter the hypothalamus through the tanycytes [ 56 ]. Astrocytes, located at the interface between blood vessels and neurons, can function as metabolic sensors. This physical location enables them to modulate glucose fluxes between the periphery and the central nervous system [ 57 ]. Hence, dynamic tanycyte–neuron interactions and astrocytes orchestrate the ongoing communication between the neuroendocrine axis and the periphery [ 58 ]. Whereas the precise role of tanycytes in PCOS is indeterminate, these cells likely allow leptin, ghrelin, and AMH access to GnRH neurons.
B-1. Preclinical models
Numerous studies have described the development of neuroendocrine features reminiscent of PCOS following prenatal androgen exposure in rodents, sheep, and rhesus macaques [ 59–61 ]. Prenatal androgen exposure during early gestation (late first to second trimester) increased LH and androgen secretion in female rhesus monkeys [ 59 ]. Prenatally androgenized (PNA) female mice showed increased γ -aminobutyric acid (GABA)ergic transmission to GnRH neurons by 3 weeks of age, suggesting that prenatal androgen treatment affected neuronal development [ 62 ]. Questions regarding prenatal imprinting of the neuroendocrine components of the HPO axis persist.
C. Valproate and HPO Axis Function
Valproic acid (VPA), a branched short-chain fatty acid derived from valeric acid, is used to treat epilepsy, bipolar disorders, and prevent migraine headaches. VPA increases GABA levels by interfering with GABA degradation pathways [ 63 ]. GnRH neurons express both GABA A and GABA B receptors, implicating GABA signaling in the regulation of GnRH secretion. Signaling through the GABA A receptor can elicit an excitatory effect on GnRH neurons [ 64 ].
Women treated with VPA can develop PCOS-like symptoms. Lean women with PCOS had significantly higher CSF GABA concentrations compared with eumenorrheic lean control women; the women with PCOS also demonstrated increased LH pulse amplitude and LH pulse frequency on frequent blood sampling [ 65 ]. These clinical observations suggest that GABA signaling could influence the neuroendocrine changes associated with PCOS such as LH pulse frequency.
C-1. Preclinical models
PNA mice models have enabled investigation regarding the consequences of prenatal androgen exposure. In an elegant series of experiments, Silva et al. [ 66 ] demonstrated increased GABA synaptic input in prepubertal PNA mice. Their observations suggest that prenatal androgenization is associated with prenatal enhanced GABAergic structural wiring input onto GnRH neurons, that these changes are reversible with long term antiandrogen treatment, and that these structural changes precede postpubertal development of PCOS features [ 66 ].
D. Insulin Resistance, Hyperinsulinemia, and the β -Cell
The phenotype of female patients with insulin receptor gene mutations includes insulin resistance (IR), compensatory hyperinsulinemia, and hyperandrogenism [ 67 ]. Although IR and hyperinsulinemia are commonly detected in women with PCOS, insulin receptor gene mutations are extremely rare among women with PCOS.
Women with PCOS have intrinsic IR independent of the extent of obesity and magnitude of androgen concentrations [ 68 ]. Even lean women with PCOS manifest IR; increasing body mass index (BMI) exacerbates IR [ 69 ]. Normal-weight adolescent girls with PCOS have peripheral IR, increased liver fat, and muscle mitochondrial dysfunction compared with normal-weight girls [ 70 ].
Insulin is the hormone primarily responsible for glucose homeostasis and lipogenesis. In addition to its effects on carbohydrate, fat, and protein metabolism, insulin functions as a mitogenic hormone. Insulin actions are mediated by insulin receptors, which are found in numerous tissues of the HPO axis. In steroidogenic tissues such as the ovary and the adrenal cortex, insulin potentiates the cognate trophic hormones to promote steroidogenesis. The compensatory hyperinsulinemia associated with IR provokes excessive ovarian/adrenal androgen secretion and decreases hepatic SHBG synthesis with the net result of increasing circulating testosterone concentrations. This leads to the paradox of insulin signaling in PCOS; liver, skeletal muscle, and adipose tissue exhibit IR, whereas steroid-producing tissues and the pituitary retain insulin sensitivity [ 71 , 72 ]. This paradox is illustrated by differences in insulin actions in granulosa–lutein cells obtained from women with anovulation with PCOS; insulin-stimulated glucose uptake is impaired whereas insulin-stimulated progesterone production is preserved [ 73 ].
The central role of compensatory hyperinsulinemia has been established by improved clinical features with insulin-sensitizing medications and weight loss. The transient IR and hyperinsulinemia typical of early puberty may kindle the factors associated with development of PCOS [ 74 , 75 ].
The prevalence of the metabolic syndrome defined as obesity, hypertension, dyslipidemia, and hyperglycemia is approximately threefold higher in women with PCOS [ 76 ]. Although a consensus definition of metabolic syndrome in adolescents is lacking, published pediatric criteria are based on adult criteria and include a combination of elevated triglyceride concentration, elevated low high-density lipoprotein cholesterol concentration, fasting blood glucose ≥110 mg/dL, increased waist circumference, and hypertension for age [ 77 ]. A meta-analysis suggested that although IR is likely a common factor linking the metabolic and reproductive features of PCOS, the metabolic and reproductive features develop through independent mechanisms [ 78 ]. One relatively consistent finding is that obesity exacerbates the symptoms of PCOS, especially regarding the risk for development of T2D and the metabolic syndrome [ 76 ].
Primary hyperinsulinemia can precede the development of peripheral tissue IR. It is beyond the scope of this review to discuss arguments supporting the opposing viewpoints, that is, primary IR vs primary hyperinsulinemia [ 79 ]. Importantly, numerous genetic and epigenetic factors, nonheritable prenatal and extrauterine environmental influences, and varying adaptations to nutrient excess likely contribute to the development of IR and hyperinsulinemia.
D-1. Preclinical models
Preclinical data show β -cell dysfunction associated with hyperinsulinemia in monkeys and sheep prenatally exposed to androgens [ 80 , 81 ].
E. Obesity, the Adipocyte, and Nutrient Excess
Overweight and obesity are common among adolescent girls and adult women with PCOS. In response to nutrient excess, adipocytes can enlarge (hypertrophy) or form new adipocytes (hyperplasia). According to the adipose tissue expandability hypothesis, adipocyte hypertrophy establishes a microenvironment characterized by hypoxia, proinflammatory cytokine secretion, free fatty acid “spillover,” macrophage invasion, and IR [ 82 ]. IR decreases suppression of adipocyte lipolysis, resulting in increased serum free fatty acids and triglycerides, ultimately leading to increased hepatic de novo lipogenesis and hyperlipidemia [ 83 ]. Another consequence is increased fat storage in skeletal muscle, liver, and pancreas because the adipose tissue capacity to store lipid is exceeded. In the liver, ectopic fat storage is labeled hepatic steatosis, which can develop into nonalcoholic fatty liver disease [ 84 ].
White adipose tissue has several distinct locations, that is, visceral and subcutaneous. Partitioning of fat among different storage sites influences metabolic consequences: increased abdominal fat is associated with greater risk for dysglycemia and cardiovascular disease. Investigation of normal-weight women with PCOS showed increased total abdominal fat mass due to preferential deposition of intra-abdominal fat with an increased population of small subcutaneous abdominal adipocytes [ 85 ]. In a pilot study involving normal-weight women with PCOS, subcutaneous adipose IR correlated with serum androgen concentrations and the percentage of small subcutaneous abdominal adipocytes. These data support the hypothesis that expansibility of the subcutaneous abdominal adipose depot is limited and unable to expand sufficiently to meet the metabolic needs for most normal-weight women with PCOS [ 86 ]. Emerging pilot data in adolescent girls with PCOS showed that reduction of visceral fat improved menstrual irregularity [ 87 ].
In a small cross-sectional study, girls related to women with PCOS showed higher 17-hydroxyprogesterone concentrations, decreased insulin sensitivity, and decreased insulin-induced suppression of nonesterified fatty acid concentrations compared with healthy control girls. These findings suggest onset of adipocyte dysfunction, IR, and possible lipotoxicity among girls aged ∼9 to 15 years [ 88 ]. In another small study using frequently sampled IV glucose tolerance tests, the authors reported early β -cell dysfunction in first-degree female relatives with overweight/obesity of women with PCOS compared with control girls with overweight/obesity [ 89 ]. Small sample sizes limit the conclusions that can be drawn from these studies. Nevertheless, the studies hint that β -cell function and insulin sensitivity may differ beginning in childhood and early adolescent years among girls “destined” to develop PCOS.
Mismatches between prenatal and postnatal weights have led to the advance of the developmental origins of disease hypothesis [ 90 ]. The longitudinal prospective population-based study (Northern Finland Birth Cohort Study) found that women with PCOS had lower birth weights, experienced adiposity rebounds at younger ages, and had higher subsequent BMI values [ 91 ]. These findings are consistent with the concept that a mismatch between prenatal weight and postnatal weight gain is associated with increased risk for PCOS, ectopic fat storage, and hepatic steatosis [ 92–94 ].
Adipose tissue expresses enzymes that activate and inactivate androgen precursors. The enzyme aldo-ketoreductase type 1C, encoded by the AKR1C3 gene, is expressed in adipose tissue and converts the preandrogen androstenedione to testosterone. Additionally, the enzyme 5 α -reductase type 1, encoded by the SRD5A1 gene, converts testosterone to DHT and is expressed in adipose tissue. A deep in vivo metabolic phenotyping study showed increased AKR1C3 and decreased SRD5A1 mRNA expression in subcutaneous fat of women with PCOS [ 95 ]. Activation appears to regulate adipocyte proliferation and differentiation, insulin sensitivity, adipokine signaling, and lipid metabolism [ 96 ]. Using a human preadipocyte cell line, both testosterone and DHT increased de novo lipogenesis in the absence of insulin [ 95 ], whereas pharmacologic inhibition of AKR1C3 activity prevented androgen-mediated adverse effects on adipocyte lipogenesis. Using this model system, insulin increased AKR1C3 expression. Based on these data, O’Reilly et al. [ 95 ] proposed the existence of a vicious cycle linking adipocyte androgen biosynthesis and adipocyte lipid accumulation to IR and hyperinsulinemia.
Another situation demonstrating androgen effects on lipid metabolism was described in girls with obesity with and without PCOS. Girls with obesity with PCOS compared with those without PCOS demonstrated decreased lipid mobilization, diminished fat oxidation, and impaired ability to switch from lipid to carbohydrate oxidation during insulin stimulation (metabolic inflexibility) [ 97 ].
E-1. Preclinical models
In a treatment paradigm comparing a high-fat diet with/or without testosterone treatment in rhesus monkeys, the combination of a high-fat diet and testosterone treatment accelerated development of white adipose tissue dysfunction [ 98 ].
F. Developmental Hypothesis/Fetal Origins
The developmental theory of PCOS proposes that exposure of the female fetus to elevated androgen concentrations contributes to the development of PCOS. Potential mechanisms include effects on steroidogenesis, insulin signaling, pancreatic β -cell function, hypothalamic–pituitary organization, neuroendocrine secretory patterns, and epigenetic modifications [ 99 ].
Fetal, neonatal, prepubertal, and/or pubertal ovaries may be genetically predisposed to increased androgen secretion [ 100 , 101 ]. Women with classical CAH often develop a secondary PCOS phenotype; it is unclear whether this reflects prenatal imprinting of the hypothalamus and GnRH pulse generator or androgen effects on the ovary [ 102 ]. Available data support the hypothesis that prenatal androgen exposure programs the neuroendocrine, metabolic, and reproductive manifestations of PCOS [ 103 ]. Women with PCOS typically have higher androgen concentrations than do women without PCOS. One report involving 23 mothers self-reporting PCOS and 277 women reporting no PCOS indicated increased anogenital differences, a marker of prenatal androgen exposure, in daughters of women with PCOS [ 104 ]. How the fetus is exposed to androgen excess when placental aromatase and maternal SHBG limit fetal exposure to maternal androgens remains an enigma.
F-1. Preclinical models
Preclinical models involving androgen exposure in rodents, sheep, and NHPs recapitulate features of PCOS. Impaired adipocyte differentiation has been demonstrated in NHP models [ 105 ]. Among prenatally androgenized NHPs, when the capacity of subcutaneous adipocytes to store fat is exceeded, excess free fatty acids may be deposited in ectopic locations such a liver and muscle; consequences of ectopic fat deposition may include impaired tissue hypoxia, inflammation, and IR [ 106 ]. Curiously, transient pancreatic dysfunction manifested by hypoglycemia, an increased number of β -cells, small islets, and relative hyperinsulinemia have been observed in this NHP model of early gestational androgen exposure [ 80 ]. Early pubertal NHP treated with testosterone and a “Western style diet” with increased fat content showed increased larger visceral adipocytes, greater IR, and ectopic fat storage [ 106 ].
G. Microbiome
Bacteria, archaea, fungi, and viruses comprise the microbial community or microbiome of the gastrointestinal tract. These organisms play roles in fermentation of dietary fiber, bile acid metabolism, host defense, and modulation of metabolism. It has been suggested that the gut microbiome influences development of nonalcoholic fatty liver disease and is associated with insulin sensitivity [ 107 , 108 ]. Sex and sex steroids modulate the composition of the gut microbiome. Women are reported to show greater α -diversity. α -Diversity represents the number of species, and β -diversity indicates similarity between samples. Decreased α -diversity has been described in women with PCOS [ 109 , 110 ]. Numerous questions remain to be answered regarding the functional relationships, if any, between sex steroids, metabolic dysregulation, and the gut microbiome [ 111 ]. To the best of our knowledge, no data for adolescents are available.
H. Genetics
Twin studies suggest that the hereditability is ∼70% [ 112 ]. The few identified genetic loci explain only a modest proportion of estimated hereditability. GWASs involving women of Han Chinese and European origins have identified at least 16 susceptibility loci for PCOS [ 113–116 ]. Several genetic variants are similar in both Han Chinese and European populations, implying that PCOS is an ancient disease [ 117 ]. Several novel loci have recently been identified [ 118 ]. A meta-analysis showed that identified loci are linked to genes plausibly associated with the metabolic and reproductive characteristics of PCOS [ 118 ]. Linkage disequilibrium score regression analysis demonstrated genetic correlations with metabolic traits, that is, fasting insulin, lipid levels, and PCOS. With the exception of the GATA4/NEIL2 locus, the genetic architecture did not differ whether National Institutes of Health or Rotterdam criteria were used to diagnose PCOS [ 118 ]. Genes involved in HPO axis function, that is, LHCGR , FSHR , and FSHB , were identified in these GWASs implicating gonadotropins in the pathophysiology of PCOS [ 115 ]. Using family-based quantitative trait meta-analysis, rare DENND1A variants were associated with metabolic and reproductive traits in PCOS families; these data are consistent with the hypothesis that complex disorders such as PCOS are associated with genetic variations in noncoding regions [ 119 ]. Epigenetic modifications such as changes in methylation and miRNAs offer another level of regulation affecting the PCOS phenotype. Epigenetic variants have been reported for adipose tissue and muscle [ 120 , 121 ].
The classic features of PCOS include clinical or biochemical hyperandrogenism, oligomenorrhea or amenorrhea associated with chronic anovulation, and polycystic ovary syndrome morphology [ 122 ]. The current consensus is that use of the Rotterdam criteria is appropriate for adult women. For diagnosis of PCOS, women must fulfill two of the three characteristics: oligo-ovulation or anovulation, clinical and/or biochemical hyperandrogenism, or polycystic ovary morphology on ultrasound with exclusion of other disorders. The 2012 National Institutes of Health–sponsored Evidence-Based Methodology PCOS Workshop categorized PCOS into four phenotypes as follows: phenotype A, hyperandrogenism, ovulatory dysfunction, and polycystic ovary morphology; phenotype B, hyperandrogenism and ovulatory dysfunction; phenotype C, hyperandrogenism and polycystic ovary morphology; and phenotype D, ovulatory dysfunction and polycystic ovary morphology [ 123 , 124 ].
However, delineating appropriate diagnostic criteria for PCOS among adolescent girls has been problematic because irregular menses, cystic acne, mild hyperandrogenism, and multifollicular ovarian morphology occur during normal pubertal maturation. These similarities between normal pubertal development and the clinical features associated with PCOS confound the diagnosis in adolescent girls ( Table 1 ) [ 125–127 ]. Similar to the evaluation of adult women, other disorders associated with irregular menses and/or hyperandrogenism need to be excluded. These disorders include CAH, typically nonclassic 21-hydroxylase deficiency, androgen-secreting tumors, thyroid dysfunction, hyperprolactinemia, Cushing syndrome, exogenous use of steroid hormones/androgens, or severe IR syndrome [ 128 , 129 ].
Definition of Irregular Menses in Adolescent Girls
[Adapted from: Teede HJ, Misso ML, Costello MF, Dokras A, Laven J, Moran L, Piltonen T, Norman RJ; International PCOS Network. Recommendations from the international evidence-based guideline for the assessment and management of polycystic ovary syndrome. Fertil Steril 2018;110(3):364–379.].
With reactivation of the GnRH pulse generator, increased gonadotropin secretion stimulates ovarian estrogen secretion and follicular development. Estrogen promotes uterine growth and endometrial proliferation; endometrial estrogen exposure eventually culminates in vaginal withdrawal bleeding and menarche. A longitudinal study found that the median age at menarche for American girls was 12.25 years, with lower menarcheal ages in black and Hispanic girls compared with white and Asian girls [ 130 ]. By age 15 years, 98% of girls will have experienced menarche [ 131 ].
Contemporary understanding is that it takes 3 to 4 years postmenarche for adult menstrual cyclicity to mature. By the third year after menarche, 10 or more menses occur annually in 90% of adolescent girls [ 132 ]. Approximately 41% of girls have achieved ovulatory cycles by the fourth gynecologic year [ 133 ]. Importantly, ovulation may occur despite irregular menses [ 134 ].
Currently, evidence-based data regarding the first gynecologic year are limited and are largely derived from studies published prior to 2000. A 2018 systemic review of menstrual patterns during the first gynecologic year concluded that menstrual and ovulatory patterns are diverse during this time period. In 22 studies involving >2000 adolescents, frequent menstrual bleeding (<21 days) occurred in 23% and prolonged menstrual bleeding (>30 to 45 days) occurred in at least 33% [ 135 ]. A pilot study entailing serial hormone concentrations and ultrasound studies in ovulatory postmenarcheal girls revealed lower steroid (estrogen and progesterone) concentrations, slower dominant follicle growth rate, and longer follicular phases compared with adult women; these data suggest that coordinated development of all components of the HPO axis may take up to 5 years postmenarche [ 136 , 137 ].
Oligomenorrheic adolescents tend to have persistent oligomenorrhea [ 138 , 139 ]. Secondary amenorrhea for >90 days is uncommon and warrants additional consideration. Girls presenting with primary amenorrhea at ages 15 to 16 years merit further evaluation.
B. Hyperandrogenism
Hirsutism, defined as excessive terminal hair growth in male pattern distribution in women, is the primary clinical sign of hyperandrogenism. The modified semisubjective Ferriman–Gallwey scoring system is one widely used approach [ 140 , 141 ]. The extent of the clinical features of hyperandrogenism represents the interactions between circulating androgen concentrations, local androgen concentrations, and sensitivity of the pilosebaceous unit/hair follicle to androgens. The severity of hirsutism does not correlate with circulating androgen concentrations. Ethnic and genetic variations influence the development of hirsutism [ 141 ]. Depending on ethnicity, a modified Ferriman–Gallwey score ≥4 to 6 indicates hirsutism [ 125 ]. Other cutaneous signs of androgen excess include severe cystic acne and male pattern baldness.
Biochemical hyperandrogenism is confirmed by documentation of elevated serum androgen concentrations. One caveat is the importance of measuring androgens using high-quality assays such as liquid chromatography–tandem mass spectrometry or extraction/chromatography immunoassays [ 142 ]. Calculated free testosterone, free androgen index, calculated bioavailable testosterone, androstenedione, and DHEAS may provide helpful information. Testosterone determinations are confounded by several problems, including inadequate assay sensitivity to accurately measure low concentrations, limited evidence-based normal ranges, assay interference due to other steroid molecules or SHBG, and technical aspects of the assay methodology. In view of these constraints, the Canadian Laboratory Initiative in Pediatric Reference Intervals (CALIPER) project has developed sensitive and accurate liquid chromatography–tandem mass spectrometry methodology to simultaneously measure eight steroids [ 143 ]. Measuring 11-oxo-androgens shows promise as a method to assess for hyperandrogenism [ 144 , 145 ].
C. Polycystic Ovary Morphology
Polycystic ovary morphology (PCOM) is defined as enlarged ovaries with increased stroma and more small peripheral cysts. The Androgen Excess–PCOS Society Task Force recommended that PCOM is defined as ≥20 follicles per ovary using a transvaginal probe and high-resolution technology (transducer frequency ≥8 MHz) [ 146 ]. However, assessment of ovarian morphology is difficult in the adolescent girl because the increased gonadotropin stimulation leads to increased ovarian volume and follicular growth, giving rise to the appearance of multifollicular ovaries in adolescent girls. Additionally, use of transvaginal probes are problematic in adolescent girls. PCOM is an inconsistent finding in adolescent girls and is not associated with anovulation or metabolic abnormalities [ 147 ]. Hence, ovarian ultrasounds are unnecessary in adolescent girls.
D. Evaluation and Diagnosis
The approach to the evaluation of a girl with signs and symptoms suggestive of PCOS begins with a thorough history, including detailed family history and complete physical examination. The individualized laboratory evaluation typically includes thyroid function studies as well as the determination of prolactin, total testosterone, androstenedione, SHBG, DHEAS, and 17-hydroxyprogesterone concentrations. Direct free testosterone assays should be avoided due to inadequate sensitivity, accuracy, and reproducibility of available assays. Fasting glucose, HbA1c, and lipid concentrations should be determined. Ideally, the blood sample should be obtained prior to 8:30 am . If CAH is a diagnostic possibility, an ACTH stimulation test can be obtained. The cut point of a basal 17-hydroxyprogesterone >200 ng/dL has been suggested as the threshold for performing ACTH stimulation tests [ 148 ]. Nevertheless, when the clinical picture is highly suggestive of a steroidogenic enzyme deficiency, an ACTH stimulation test might be warranted. Adrenal and pelvic imaging may be considered depending on the clinical information, physical examination, and initial laboratory data.
AMH concentrations are often elevated in women with PCOS. AMH concentrations reflect ovarian reserve and are correlated with the number of growing follicles [ 149 ]. Although it is premature to use AMH concentrations to diagnose PCOS, AMH concentrations have been found to be elevated in nonobese girls with PCOS [ 150 , 151 ]. AMH concentrations were found to be higher in girls with obesity with PCOS compared to girls with obesity without PCOS of comparable age and pubertal status [ 152 ].
Insulin resistance, hyperinsulinemia, and obesity are commonly identified in women with PCOS. However, with the exception of a single publication, none of the current definitions, recommendations, or guidelines includes IR and/or hyperinsulinemia as a diagnostic feature [ 153 ].
Hence, the diagnosis of PCOS can be considered for the adolescent girl with persistence of oligoamenorrhea for 3 to 4 years postmenarche with clinical and/or biochemical hyperandrogenism after exclusion of other disorders associated with irregular menses or hyperandrogenism. When oligomenorrhea has not persisted for >2 years, these girls can be considered to be “at risk” for PCOS and require longitudinal evaluation to assess for ongoing features of PCOS. Deferred diagnosis attempts to avoid overdiagnosis with its potential for premature labeling, anxiety, and unnecessary interventions. Nevertheless, diagnostic labeling needs to be balanced with the patient’s desire for a diagnosis and specific therapeutic interventions [ 125–127 , 154 , 155 ].
Adolescents presenting with PCOS features, before the diagnosis is confirmed, often require management of their symptoms [ 125–127 ]. The management of adolescents with a clear diagnosis of PCOS should include education about the condition and lifestyle interventions. The interventions can be individualized to target the foremost complaints and symptoms. Interventions include metformin, combined oral contraceptive pills (COCPs), spironolactone, and local treatments for hirsutism and acne. Management should also include management of comorbidities, regular follow-up, and a plan for transition to adult care providers.
A. Education and Counseling
Education and counseling about the condition is very important. The explanation and discussion of PCOS should be culturally sensitive as well as appropriate, comprehensive, and tailored to the individual [ 125 ]. This discussion should use an empathetic approach, promote self-care, and highlight peer support groups, which are available in multiple countries ( www.pcoschallenge.org/ , www.verity-pcos.org.uk/ , and www.facebook.com/PCOSAustralia/ ). Counseling about fertility concerns is important, as adolescents with PCOS are more concerned than theirs peers about future fertility after diagnosis [ 156 ].
B. Lifestyle Interventions
Healthy lifestyle interventions must be incorporated in the management plan of all adolescents with PCOS [ 125–127 ] because a large proportion of these adolescents are overweight/obese or are at risk for gaining excessive weight [ 157 ]. Lifestyle interventions comprise multiple components, including healthy diets, physical activity, decreased sedentary behaviors, and behavioral strategies [ 158 ]. The interventions should also include the family, as parents’ involvement and their readiness to change affect adolescent outcomes [ 159 , 160 ]. Engagement and adherence to lifestyle interventions can be improved by management of psychological factors such as anxiety, body image concerns, and disordered eating, which are common in adolescents [ 125 , 161 ]. Two systematic reviews of lifestyle interventions in women with PCOS show improvements in weight, hyperandrogenism, and IR [ 162 , 163 ]. Lifestyle interventions in adolescents with PCOS have shown additional improvements in quality of life [ 160 , 164 , 165 ].
Limited data are available regarding the specific type of diet to achieve weight loss in PCOS [ 125 ]. Five randomized controlled trials (RCTs) have evaluated diets in the management of adolescents with overweight/obesity with PCOS, with only three that evaluated diet as a single intervention [ 160 , 164 , 166–168 ]. A low-carbohydrate diet (20 to 40 g/d) and a hypocaloric diet (<40 g of fat per day) during 12 weeks improved weight and menstrual irregularities with no difference between the diets. Similarly, both low–glycemic load and low-fat diets during 6 months improved weight with no difference between diets [ 168 ]. A low-energy diet compared with a healthy diet for 6 months was associated with weight loss, more regular menses, and decreased hirsutism [ 167 ]. Nutrition education in addition to exercise training and behavioral therapy for 12 months resulted in weight loss, as well as improvement of menstrual irregularities and androgen levels in adolescents with obesity and PCOS [ 160 ].
Physical activity of longer duration, frequency, and intensity results in better maintenance of health. Importantly, moderate to vigorous physical activity for at least 60 minutes per day is associated with better physical and psychosocial health in children and adolescents [ 169 ]. Sixty minutes of moderate to vigorous physical activity at least 3 times a week should be encouraged for the prevention of weight gain and maintenance of health in PCOS [ 125 , 170 ]. Exercise interventions can also improve cardiometabolic risk factors in women with PCOS [ 171 ]. Alternative exercise activities such as yoga for 12 weeks can also improve PCOS symptoms during adolescence [ 172 ]. Limiting sedentary behaviors such as watching television and the use of tablets, computers, and/or mobile phones to 2 h/d is advised for adolescents and relates to better health [ 173 ].
Data regarding behavioral interventions in adolescents with PCOS are limited [ 125 ]. However, family therapy in addition to other lifestyle interventions show beneficial effects on adolescent PCOS symptoms, and a small open trial shows that cognitive behavior therapy improved depressive symptoms [ 160 , 174 ].
Prevention of weight gain and effective weight management is important in adolescent PCOS, as obesity exacerbates metabolic and psychological comorbidities of PCOS [ 175 , 176 ]. Additionally, weight loss strategies up to 7% of body weight have resulted in improving menstrual irregularity and testosterone levels [ 164 , 167 ]. There are limited data for the use of weight loss medications in adolescents.
C. Metformin
Metformin is the single most studied insulin sensitizer in PCOS. It is commonly used in adolescents 15 to 19 years of age despite being “off label” for this indication [ 177 ]. Additionally, according to the recent international evidence-based guidelines for assessment and management of PCOS, “The use of metformin in addition to lifestyle could be considered in adolescents with a clear diagnosis of PCOS or with symptoms of PCOS before the diagnosis is made” [ 125 ].
A meta-analysis of metformin use with and without lifestyle changes in PCOS (including two RCTs in adolescents [ 164 , 178 ]) showed beneficial effects on BMI and menstrual cycles [ 164 , 178 , 179 ]. There have been multiple observational studies and six RCTs evaluating the effect of metformin on a total of 275 adolescents with PCOS. These studies have demonstrated short-term beneficial effects mostly in adolescents with overweight/obesity [ 164 , 180–183 ]. Metformin doses used ranged from 1000 to 2000 mg daily with the major side effect being mild gastrointestinal distress. Limitations are that the frequency of side effects and adherence to medications have not been fully reported. Side effects can be reduced by starting metformin at a lower dose with slow increments and the use of extended release preparations. RCTs were mostly of 6-month duration; only one study lasted 24 months, and no longer-term studies have been reported.
Metformin at a dose of 1700 to 2000 mg/d is associated with greater improvement of BMI, and COCPs are associated with improvement in menstrual irregularity and acne according to a meta-analysis of metformin vs oral contraceptives in adolescents with PCOS and including four RCTs (170 adolescents) [ 164 , 180–184 ]. Both metformin and oral contraceptives had similar beneficial effects on hirsutism, triglycerides, and high-density lipoprotein cholesterol, but the estimates of effect were derived from low-quality evidence involving small studies [ 184 ]. Meta-analyses including larger number of RCTs in women with PCOS showed limited or no benefit of insulin sensitizers on hirsutism [ 185 , 186 ].
Metformin also can be used in addition to COCPs, especially in adolescents with PCOS and BMI ≥25 kg/m 2 , as well as high–metabolic risk groups such as certain ethnicities and individuals at increased risk of type 2 diabetes [ 125 ].
COCPs (estrogen and progestin preparations) should be considered for management of menstrual irregularity and/or clinical hyperandrogenism in adolescents with a clear diagnosis of PCOS and in adolescents at risk of PCOS before the diagnosis is confirmed according to the recent international evidence-based guidelines [ 125 ]. There are limited evidence-based data regarding specific types or doses of progestins, estrogens, or combinations of COCPs for management of PCOS in adolescents and women, but the lowest effective estrogen dose (20 to 30 µg of ethinylestradiol) should be considered [ 125 ]. Contraindications such as thromboembolism risk should be assessed when prescribing COCPs by obtaining thorough medical histories of the patient and her family. In most instances, 35 µg of ethinylestradiol plus cyproterone acetate preparations should not be considered first line in PCOS [ 125 , 187 ]. Duration of treatment has not been evaluated beyond 24 months in adolescents with PCOS. However, COCPs have been used for contraception in longer periods of time.
COCPs improve menstrual irregularity in adolescents with PCOS [ 164 , 180–184 ]. COCPs should be also offered when contraception is required and/or medical treatment of hirsutism or acne is needed [ 184 ]. When no contraception is required, menstrual irregularity alone can also be managed with cyclical medroxyprogesterone acetate (10 mg per day for 10 days) [ 188 , 189 ]. This can be offered when there is a desire to have fewer menstrual cycles and/or a preference for not taking daily medications or being on COCPs due to cultural reasons.
E. Management of Hirsutism
Acknowledgment of the significance of the hirsutism, irrespective of the severity, for a particular adolescent is important when offering treatment options as well as understanding expectations of the treatment [ 125 ]. Long-term commitment is required for any topical and/or medical interventions. More severe hirsutism may require a combination of strategies. Current available therapies have been mostly evaluated in women and include physical hair removal methods, topical medications, light-based therapies, COCPs, and antiandrogens [ 190–192 ].
Physical hair removal methods include waxing, shaving, chemical epilation, plucking, bleaching, and electrolysis. All but electrolysis are temporary hair removal methods, easily available and commonly used by adolescents even before they are evaluated for PCOS. There have been no RCTs evaluating these methods. Electrolysis is a permanent hair removal method, as it causes destruction of hair bulb, but it requires an experienced technician and can cause scaring and pigmentation changes [ 193 ].
Topical medications such as 13.9% eflornithine cream, an irreversible inhibitor of ornithine decarboxylase, affects hair follicle growth and differentiation and can improve mild facial hirsutism in women with mild skin irritation [ 194 , 195 ].
Professional light-based therapies include lasers (alexandrite, diode, and neodymium-doped yttrium aluminum) and intense pulsed light. These light therapies provide wavelengths of 600 to 1100 nm that are absorbed by the melanin in the hair and destroy the hair. This approach provides a prolonged solution for hirsutism after multiple treatments. The light can also be absorbed by epidermal melanin, which is greater in darker skinned individuals, increasing the risk of blisters, dyspigmentation, and scarring [ 196 ]. The neodymium-doped yttrium laser has longer wavelengths, which is less absorbed by epidermal melanin of darker skinned individuals, decreasing side effects. Light-based therapies should be the first line of treatment of localized hirsutism [ 125 , 126 ]. Laser treatment was associated with a 50% reduction of hair at 6 months after treatment with mild side effects such as pain, skin redness, and perifollicular edema [ 197 ]. Uncommon side effects include burns, blisters, hyperpigmentation/hypopigmentation, and scarring that can be reduced by topical anesthetic creams prior to treatment and by cooling mechanisms after treatment. Sun exposure should be avoided before and after treatment. Improvement of hirsutism with laser has been associated with improvement in quality of life, anxiety, and depression in young women with PCOS [ 198 , 199 ].
Light-based home-use devices are also available and approved by the US Food and Drug Administration. These devices provide less optical energy and should be carefully used to avoid injuries to skin and eyes [ 200 , 201 ]. Fewer RCTs have evaluated the efficacy of these devices [ 202 ].
Hormonal therapies should be considered in moderate or severe forms of hirsutism and include COCPs and antiandrogens [ 192 ]. COCPs alone improve hirsutism in adolescents with PCOS [ 184 ]. Estrogens in the COCPs decrease free androgens by increasing hepatic production of SHBG and decrease ovarian and adrenal androgen production by suppressing LH levels [ 203 ]. Progestins in the COCPs also have some antiandrogenic properties by blocking the AR and inhibiting 5 α -reductase activity. A small RCT involving adolescents with PCOS showed no difference in hirsutism improvement when two COCPs were compared during 12 months (30 µg of ethinyl estradiol and 0.15 mg of desogestrel vs 35 µg of ethinyl estradiol and 2 mg of cyproterone acetate) [ 204 ]. However, cyproterone acetate is not available in the United States.
Spironolactone, cyproterone acetate (which can be part of COCPs), and flutamide are antiandrogens that have been evaluated and used to treat hirsutism in women [ 191 ]. Spironolactone is an aldosterone antagonist that blocks the AR. It should be used after 6 months of COCPs; monitoring for side effects such as volume depletion and electrolyte disturbances should be explained and performed [ 125 , 205 ]. The starting dose for spironolactone is ∼25 mg/d. Subsequently, doses can range from 100 to 200 mg/d divided in two doses. Flutamide at a dose of 250 to 500 mg/d divided in two doses during 12 months has shown beneficial effects on hirsutism in women, but there are no RCTs evaluating the effect of flutamide alone or in combination with COCPs in adolescents. Low doses of flutamide (125 mg/d) in combination with metformin have been used in adolescents with ovarian hyperandrogenism [ 206 ]. Flutamide has been associated with severe side effects such as liver toxicity [ 191 , 207 ]. Finasteride is a topical medication that inhibits 5 α -reductase that should be avoided in adolescents, as data are very limited even among adult women [ 190 ].
Antiandrogens alone could be considered to treat hirsutism or alopecia when COCPs are contraindicated or poorly tolerated. However, antiandrogens must be used with effective contraception in sexually active adolescents to avoid fetal undervirilization [ 125 , 126 ]. The combination of COCPs and antiandrogens is superior for management of hirsutism [ 186 ].
F. Management of Acne
Treatment will be guided by severity of acne with the following goals of treatment: reduction of sebum production, prevention of formation of microcomedones, suppression of Propionibacterium acnes , and reduction of inflammation to prevent scaring [ 208 ]. Mild acne can be managed initially with over-the-counter topical treatments such as benzoyl peroxide 0.1%/2.5% (Epiduo gel) or topical retinoids or the combination of the two agents as well as appropriate skin care. Moderate and severe forms of acne require the addition of systemic antibiotics (macrolides) for 3 or 4 months but discontinuation after new inflammatory lesions have stopped appearing [ 208 , 209 ]. COCPs can also be added for management of moderate to severe acne in adolescents [ 184 ]. Timely referral to a dermatologist should be considered when the response is poor or in severe cases, as acne has a major negative impact on adolescent psychosocial well-being.
G. Screening of Other Comorbidities
Additional comorbidities can occur in adolescents with PCOS that might be independent of overweight status. These comorbidities include impaired glucose tolerance and type 2 diabetes [ 125 , 126 , 210 , 211 ]. Additional comorbidities include decreased quality of life, depression, anxiety, eating disorders and disordered eating, and altered body image [ 192 , 212–214 ]. Identification of IR, hyperinsulinemia, and obesity galvanizes efforts to investigate and initiate treatment of associated comorbidities such as impaired glucose tolerance, type 2 diabetes mellitus, dyslipidemia, and sleep apnea. Adequate screening for comorbidities should be guided by symptoms, clinical examination, and specific personal and family risks factors. This should be followed by appropriate management to avoid further complications [ 125 , 215 , 216 ].
As per management of any adolescent, the HEEADDSS screening tool should be used (that is, H, home environment; E, education and employment; E, exercise and healthy eating; A, activity and peers; D, drugs, smoking, and alcohol; D, depression and suicide ideation; S, sexuality and sexual health; S, sleep) [ 217 ]. Prompt referral to social work, psychology, and counseling in the presence of psychosocial comorbidities is necessary, as these comorbidities will affect adherence to any interventions. Self-management strategies such as mindfulness and yoga in PCOS are emerging and require more research [ 172 , 218 ]. Contraception should be discussed in sexually active adolescents with PCOS who are not taking COCPs for PCOS.
H. Transition to Adult Care Providers
Preparation for transition to adult care will require reinforcement of education about PCOS, its comorbidities, lifestyle interventions, medical treatment, and the need of long-term follow-up [ 125 , 126 , 219 ]. Women with PCOS are best managed by multidisciplinary health care teams comprised of endocrinologists, general physicians, gynecologists, family doctors, or general practitioners. Therapeutic options should be discussed with the adolescent or emerging adult. The selection of an appropriate specialist for adult care should be based on adolescent preferences and major complaints, local availability of health care professionals or specialized clinics, health care insurance, and the possible need of fertility management in the near future.
PCOS is a complex disorder involving multiple organ systems with onset during the early pubertal years ( Fig. 1 ). The list of factors involved in the pathophysiology continues to expand, with accruing evidence indicating that hyperandrogenism is a pivotal factor affecting multiple tissues [ 220 , 221 ]. GWASs have identified genes common to both Han Chinese and white populations that are involved in neuroendocrine, metabolic, and reproductive pathways [ 118 ]. Data obtained from animal models have consistently implicated testosterone as an important factor in the pathogenesis of PCOS. The important contributions of ectopic fat storage and adipocyte androgen biosynthesis are emerging. Promising clinical and preclinical data point toward neuroendocrine involvement with supporting roles for GABA signaling and neuronal ARs.
At this time, an individualized treatment plan can be developed for the adolescent girl with features of PCOS. Attention to the history, physical examination, and laboratory data is important to identify adolescent girls at risk to develop PCOS. Whereas deferring diagnostic labeling may be appropriate, treatment of clinical features and comorbidities is vital to the health and self-esteem of these patients. One future goal includes prevention through timely identification of at-risk prepubertal and early pubertal girls through lifestyle interventions.
Financial Support: This work was supported in part by the Australian National Health and Medical Research Council Centre for Research Excellence scheme in the origins, outcomes, and optimal management of PCOS (Grant APP1078444 to A.S.P.).
Disclosure Summary: The authors have nothing to disclose.
Data Availability: Data sharing is not applicable to this article as no data sets were generated or analyzed during the current study.
Abbreviations:
anti-Müllerian hormone
androgen receptor
body mass index
congenital adrenal hyperplasia
combined oral contraceptive pill
dehydroepiandrosterone
dehydroepiandrosterone sulfate
FSH receptor
γ -aminobutyric acid
genome-wide association study
hypothalamic–pituitary–ovarian
insulin resistance
LH/chorionic gonadotropin receptor
nonhuman primate
polycystic ovary morphology
polycystic ovary syndrome
prenatally androgenized
randomized controlled trial
valproic acid
Hochberg Z , Feil R , Constancia M , Fraga M , Junien C , Carel JC , Boileau P , Le Bouc Y , Deal CL , Lillycrop K , Scharfmann R , Sheppard A , Skinner M , Szyf M , Waterland RA , Waxman DJ , Whitelaw E , Ong K , Albertsson-Wikland K . Child health, developmental plasticity, and epigenetic programming . Endocr Rev . 2011 ; 32 ( 2 ): 159 – 224 .
Google Scholar
Escobar-Morreale HF . Polycystic ovary syndrome: definition, aetiology, diagnosis and treatment . Nat Rev Endocrinol . 2018 ; 14 ( 5 ): 270 – 284 .
Azziz R , Carmina E , Chen Z , Dunaif A , Laven JS , Legro RS , Lizneva D , Natterson-Horowtiz B , Teede HJ , Yildiz BO . Polycystic ovary syndrome . Nat Rev Dis Primers . 2016 ; 2 ( 1 ): 16057 .
Palomba S , Daolio J , La Sala GB . Oocyte competence in women with polycystic ovary syndrome . Trends Endocrinol Metab . 2017 ; 28 ( 3 ): 186 – 198 .
Mohamed-Hussein ZA , Harun S . Construction of a polycystic ovarian syndrome (PCOS) pathway based on the interactions of PCOS-related proteins retrieved from bibliomic data . Theor Biol Med Model . 2009 ; 6 ( 1 ): 18 .
Apter D , Bützow T , Laughlin GA , Yen SS . Accelerated 24-hour luteinizing hormone pulsatile activity in adolescent girls with ovarian hyperandrogenism: relevance to the developmental phase of polycystic ovarian syndrome . J Clin Endocrinol Metab . 1994 ; 79 ( 1 ): 119 – 125 .
Lizneva D , Kirubakaran R , Mykhalchenko K , Suturina L , Chernukha G , Diamond MP , Azziz R . Phenotypes and body mass in women with polycystic ovary syndrome identified in referral versus unselected populations: systematic review and meta-analysis . Fertil Steril . 2016 ; 106 ( 6 ): 1510 – 1520.e2 .
Pache TD , Fauser BC . Polycystic ovaries in female-to-male transsexuals . Clin Endocrinol (Oxf) . 1993 ; 39 ( 6 ): 702 – 703 .
Anderson RA , McLaughlin M , Wallace WH , Albertini DF , Telfer EE . The immature human ovary shows loss of abnormal follicles and increasing follicle developmental competence through childhood and adolescence . Hum Reprod . 2014 ; 29 ( 1 ): 97 – 106 .
Gaytan F , Morales C , Leon S , Garcia-Galiano D , Roa J , Tena-Sempere M . Crowding and follicular fate: spatial determinants of follicular reserve and activation of follicular growth in the mammalian ovary . PLoS One . 2015 ; 10 ( 12 ): e0144099 .
Carlsson IB , Scott JE , Visser JA , Ritvos O , Themmen AP , Hovatta O . Anti-Müllerian hormone inhibits initiation of growth of human primordial ovarian follicles in vitro . Hum Reprod . 2006 ; 21 ( 9 ): 2223 – 2227 .
Xu J , Bishop CV , Lawson MS , Park BS , Xu F . Anti-Müllerian hormone promotes pre-antral follicle growth, but inhibits antral follicle maturation and dominant follicle selection in primates . Hum Reprod . 2016 ; 31 ( 7 ): 1522 – 1530 .
Dumont A , Robin G , Dewailly D . Anti-Müllerian hormone in the pathophysiology and diagnosis of polycystic ovarian syndrome . Curr Opin Endocrinol Diabetes Obes . 2018 ; 25 ( 6 ): 377 – 384 .
Weil SJ , Vendola K , Zhou J , Adesanya OO , Wang J , Okafor J , Bondy CA . Androgen receptor gene expression in the primate ovary: cellular localization, regulation, and functional correlations . J Clin Endocrinol Metab . 1998 ; 83 ( 7 ): 2479 – 2485 .
Harlow CR , Shaw HJ , Hillier SG , Hodges JK . Factors influencing follicle-stimulating hormone-responsive steroidogenesis in marmoset granulosa cells: effects of androgens and the stage of follicular maturity . Endocrinology . 1988 ; 122 ( 6 ): 2780 – 2787 .
Sen A , Hammes SR . Granulosa cell-specific androgen receptors are critical regulators of ovarian development and function . Mol Endocrinol . 2010 ; 24 ( 7 ): 1393 – 1403 .
Jeppesen JV , Kristensen SG , Nielsen ME , Humaidan P , Dal Canto M , Fadini R , Schmidt KT , Ernst E , Yding Andersen C . LH-receptor gene expression in human granulosa and cumulus cells from antral and preovulatory follicles . J Clin Endocrinol Metab . 2012 ; 97 ( 8 ): E1524 – E1531 .
Kristensen SG , Mamsen LS , Jeppesen JV , Bøtkjær JA , Pors SE , Borgbo T , Ernst E , Macklon KT , Andersen CY . Hallmarks of human small antral follicle development: implications for regulation of ovarian steroidogenesis and selection of the dominant follicle . Front Endocrinol (Lausanne) . 2018 ; 8 : 376 .
Franks S , Hardy K . Androgen action in the ovary . Front Endocrinol (Lausanne) . 2018 ; 9 : 452 .
Webber LJ , Stubbs S , Stark J , Trew GH , Margara R , Hardy K , Franks S . Formation and early development of follicles in the polycystic ovary . Lancet . 2003 ; 362 ( 9389 ): 1017 – 1021 .
Maciel GA , Baracat EC , Benda JA , Markham SM , Hensinger K , Chang RJ , Erickson GF . Stockpiling of transitional and classic primary follicles in ovaries of women with polycystic ovary syndrome . J Clin Endocrinol Metab . 2004 ; 89 ( 11 ): 5321 – 5327 .
Homer MV , Toloubeydokhti T , Lawson MA , Garzo G , Duleba AJ , Chang RJ . Individual 17-hydroxyprogesterone responses to hCG are not correlated with follicle size in polycystic ovary syndrome . J Endocr Soc . 2019 ; 3 ( 4 ): 687 – 698 .
Richards JS , Ren YA , Candelaria N , Adams JE , Rajkovic A . Ovarian follicular theca cell recruitment, differentiation, and impact on fertility: 2017 update . Endocr Rev . 2018 ; 39 ( 1 ): 1 – 20 .
Gilling-Smith C , Willis DS , Beard RW , Franks S . Hypersecretion of androstenedione by isolated thecal cells from polycystic ovaries . J Clin Endocrinol Metab . 1994 ; 79 ( 4 ): 1158 – 1165 .
Gilling-Smith C , Story H , Rogers V , Franks S . Evidence for a primary abnormality of thecal cell steroidogenesis in the polycystic ovary syndrome . Clin Endocrinol (Oxf) . 1997 ; 47 ( 1 ): 93 – 99 .
Nelson VL , Legro RS , Strauss JF III , McAllister JM . Augmented androgen production is a stable steroidogenic phenotype of propagated theca cells from polycystic ovaries . Mol Endocrinol . 1999 ; 13 ( 6 ): 946 – 957 .
McAllister JM , Modi B , Miller BA , Biegler J , Bruggeman R , Legro RS , Strauss JF III . Overexpression of a DENND1A isoform produces a polycystic ovary syndrome theca phenotype . Proc Natl Acad Sci USA . 2014 ; 111 ( 15 ): E1519 – E1527 .
Tee MK , Speek M , Legeza B , Modi B , Teves ME , McAllister JM , Strauss JF III , Miller WL . Alternative splicing of DENND1A , a PCOS candidate gene, generates variant 2 . Mol Cell Endocrinol . 2016 ; 434 : 25 – 35 .
Auchus RJ . The backdoor pathway to dihydrotestosterone . Trends Endocrinol Metab . 2004 ; 15 ( 9 ): 432 – 438 .
Kamrath C , Hochberg Z , Hartmann MF , Remer T , Wudy SA . Increased activation of the alternative “backdoor” pathway in patients with 21-hydroxylase deficiency: evidence from urinary steroid hormone analysis . J Clin Endocrinol Metab . 2012 ; 97 ( 3 ): E367 – E375 .
Miller WL , Auchus RJ . The “backdoor pathway” of androgen synthesis in human male sexual development . PLoS Biol . 2019 ; 17 ( 4 ): e3000198 .
Marti N , Galván JA , Pandey AV , Trippel M , Tapia C , Müller M , Perren A , Flück CE . Genes and proteins of the alternative steroid backdoor pathway for dihydrotestosterone synthesis are expressed in the human ovary and seem enhanced in the polycystic ovary syndrome . Mol Cell Endocrinol . 2017 ; 441 : 116 – 123 .
Pretorius E , Africander DJ , Vlok M , Perkins MS , Quanson J , Storbeck KH . 11-Ketotestosterone and 11-ketodihydrotestosterone in castration resistant prostate cancer: potent androgens which can no longer be ignored . PLoS One . 2016 ; 11 ( 7 ): e0159867 .
O’Reilly MW , Kempegowda P , Jenkinson C , Taylor AE , Quanson JL , Storbeck KH , Arlt W . 11-Oxygenated C19 steroids are the predominant androgens in polycystic ovary syndrome . J Clin Endocrinol Metab . 2017 ; 102 ( 3 ): 840 – 848 .
Dhayat NA , Marti N , Kollmann Z , Troendle A , Bally L , Escher G , Grössl M , Ackermann D , Ponte B , Pruijm M , Müller M , Vogt B , Birkhäuser MH , Bochud M , Flück CE ; members of the SKIPOGH Study Group . Urinary steroid profiling in women hints at a diagnostic signature of the polycystic ovary syndrome: a pilot study considering neglected steroid metabolites . PLoS One . 2018 ; 13 ( 10 ): e0203903 .
Xu J , Xu F , Lawson MS , Tkachenko OY , Ting AY , Kahl CA , Park BS , Stouffer RR , Bishop CV . Anti-Müllerian hormone is a survival factor and promotes the growth of rhesus macaque preantral follicles during matrix-free culture . Biol Reprod . 2018 ; 98 ( 2 ): 197 – 207 .
Cimino I , Casoni F , Liu X , Messina A , Parkash J , Jamin SP , Catteau-Jonard S , Collier F , Baroncini M , Dewailly D , Pigny P , Prescott M , Campbell R , Herbison AE , Prevot V , Giacobini P . Novel role for anti-Müllerian hormone in the regulation of GnRH neuron excitability and hormone secretion . Nat Commun . 2016 ; 7 ( 1 ): 10055 .
Tata B , Mimouni NE , Barbotin AL , Malone SA , Loyens A , Pigny P , Dewailly D , Catteau-Jonard S , Sundström-Poromaa I , Piltonen TT , Dal Bello F , Medana C , Prevot V , Clasadonte J , Giacobini P . Elevated prenatal anti-Müllerian hormone reprograms the fetus and induces polycystic ovary syndrome in adulthood . Nat Med . 2018 ; 24 ( 6 ): 834 – 846 .
Caldwell AS , Edwards MC , Desai R , Jimenez M , Gilchrist RB , Handelsman DJ , Walters KA . Neuroendocrine androgen action is a key extraovarian mediator in the development of polycystic ovary syndrome . Proc Natl Acad Sci USA . 2017 ; 114 ( 16 ): E3334 – E3343 .
Abbott DH , Rayome BH , Dumesic DA , Lewis KC , Edwards AK , Wallen K , Wilson ME , Appt SE , Levine JE . Clustering of PCOS-like traits in naturally hyperandrogenic female rhesus monkeys . Hum Reprod . 2017 ; 32 ( 4 ): 923 – 936 .
Lehman MN , Coolen LM , Goodman RL . Minireview: kisspeptin/neurokinin B/dynorphin (KNDy) cells of the arcuate nucleus: a central node in the control of gonadotropin-releasing hormone secretion . Endocrinology . 2010 ; 151 ( 8 ): 3479 – 3489 .
Moore AM , Coolen LM , Porter DT , Goodman RL , Lehman MN . KNDy cells revisited . Endocrinology . 2018 ; 159 ( 9 ): 3219 – 3234 .
Plant TM . Neuroendocrine control of the onset of puberty . Front Neuroendocrinol . 2015 ; 38 : 73 – 88 .
Messina A , Langlet F , Chachlaki K , Roa J , Rasika S , Jouy N , Gallet S , Gaytan F , Parkash J , Tena-Sempere M , Giacobini P , Prevot V . A microRNA switch regulates the rise in hypothalamic GnRH production before puberty [published correction appears in Nat Neurosci . 2016; 19 (8):1115] . Nat Neurosci . 2016 ; 19 ( 6 ): 835 – 844 .
Lomniczi A , Ojeda SR . The emerging role of epigenetics in the regulation of female puberty . Endocr Dev . 2016 ; 29 : 1 – 16 .
Casoni F , Malone SA , Belle M , Luzzati F , Collier F , Allet C , Hrabovszky E , Rasika S , Prevot V , Chédotal A , Giacobini P . Development of the neurons controlling fertility in humans: new insights from 3D imaging and transparent fetal brains . Development . 2016 ; 143 ( 21 ): 3969 – 3981 .
Wildt L , Häusler A , Marshall G , Hutchison JS , Plant TM , Belchetz PE , Knobil E . Frequency and amplitude of gonadotropin-releasing hormone stimulation and gonadotropin secretion in the rhesus monkey . Endocrinology . 1981 ; 109 ( 2 ): 376 – 385 .
Herbison AE . Control of puberty onset and fertility by gonadotropin-releasing hormone neurons . Nat Rev Endocrinol . 2016 ; 12 ( 8 ): 452 – 466 .
Hill JW , Elias CF . Neuroanatomical framework of the metabolic control of reproduction . Physiol Rev . 2018 ; 98 ( 4 ): 2349 – 2380 .
George JT , Kakkar R , Marshall J , Scott ML , Finkelman RD , Ho TW , Veldhuis J , Skorupskaite K , Anderson RA , McIntosh S , Webber L . Neurokinin B receptor antagonism in women with polycystic ovary syndrome: a randomized, placebo-controlled trial . J Clin Endocrinol Metab . 2016 ; 101 ( 11 ): 4313 – 4321 .
Blank SK , McCartney CR , Marshall JC . The origins and sequelae of abnormal neuroendocrine function in polycystic ovary syndrome . Hum Reprod Update . 2006 ; 12 ( 4 ): 351 – 361 .
Wakabayashi Y , Nakada T , Murata K , Ohkura S , Mogi K , Navarro VM , Clifton DK , Mori Y , Tsukamura H , Maeda K , Steiner RA , Okamura H . Neurokinin B and dynorphin A in kisspeptin neurons of the arcuate nucleus participate in generation of periodic oscillation of neural activity driving pulsatile gonadotropin-releasing hormone secretion in the goat . J Neurosci . 2010 ; 30 ( 8 ): 3124 – 3132 .
Moenter SM . Leap of faith: does serum luteinizing hormone always accurately reflect central reproductive neuroendocrine activity ? Neuroendocrinology . 2015 ; 102 ( 4 ): 256 – 266 .
Katulski K , Podfigurna A , Czyzyk A , Meczekalski B , Genazzani AD . Kisspeptin and LH pulsatile temporal coupling in PCOS patients . Endocrine . 2018 ; 61 ( 1 ): 149 – 157 .
García-Cáceres C , Balland E , Prevot V , Luquet S , Woods SC , Koch M , Horvath TL , Yi CX , Chowen JA , Verkhratsky A , Araque A , Bechmann I , Tschöp MH . Role of astrocytes, microglia, and tanycytes in brain control of systemic metabolism . Nat Neurosci . 2019 ; 22 ( 1 ): 7 – 14 .
Balland E , Dam J , Langlet F , Caron E , Steculorum S , Messina A , Rasika S , Falluel-Morel A , Anouar Y , Dehouck B , Trinquet E , Jockers R , Bouret SG , Prévot V . Hypothalamic tanycytes are an ERK-gated conduit for leptin into the brain . Cell Metab . 2014 ; 19 ( 2 ): 293 – 301 .
García-Cáceres C , Quarta C , Varela L , Gao Y , Gruber T , Legutko B , Jastroch M , Johansson P , Ninkovic J , Yi CX , Le Thuc O , Szigeti-Buck K , Cai W , Meyer CW , Pfluger PT , Fernandez AM , Luquet S , Woods SC , Torres-Alemán I , Kahn CR , Götz M , Horvath TL , Tschöp MH . Astrocytic insulin signaling couples brain glucose uptake with nutrient availability . Cell . 2016 ; 166 ( 4 ): 867 – 880 .
Prevot V , Dehouck B , Sharif A , Ciofi P , Giacobini P , Clasadonte J . The versatile tanycyte: a hypothalamic integrator of reproduction and energy metabolism . Endocr Rev . 2018 ; 39 ( 3 ): 333 – 368 .
Birch RA , Padmanabhan V , Foster DL , Unsworth WP , Robinson JE . Prenatal programming of reproductive neuroendocrine function: fetal androgen exposure produces progressive disruption of reproductive cycles in sheep . Endocrinology . 2003 ; 144 ( 4 ): 1426 – 1434 .
Sullivan SD , Moenter SM . Prenatal androgens alter GABAergic drive to gonadotropin-releasing hormone neurons: implications for a common fertility disorder . Proc Natl Acad Sci USA . 2004 ; 101 ( 18 ): 7129 – 7134 .
Abbott DH , Tarantal AF , Dumesic DA . Fetal, infant, adolescent and adult phenotypes of polycystic ovary syndrome in prenatally androgenized female rhesus monkeys . Am J Primatol . 2009 ; 71 ( 9 ): 776 – 784 .
Berg T , Silveira MA , Moenter SM . Prepubertal development of GABAergic transmission to gonadotropin-releasing hormone (GnRH) neurons and postsynaptic response are altered by prenatal androgenization . J Neurosci . 2018 ; 38 ( 9 ): 2283 – 2293 .
Johannessen CU , Johannessen SI . Valproate: past, present, and future . CNS Drug Rev . 2003 ; 9 ( 2 ): 199 – 216 .
DeFazio RA , Heger S , Ojeda SR , Moenter SM . Activation of A-type γ-aminobutyric acid receptors excites gonadotropin-releasing hormone neurons . Mol Endocrinol . 2002 ; 16 ( 12 ): 2872 – 2891 .
Kawwass JF , Sanders KM , Loucks TL , Rohan LC , Berga SL . Increased cerebrospinal fluid levels of GABA, testosterone and estradiol in women with polycystic ovary syndrome . Hum Reprod . 2017 ; 32 ( 7 ): 1450 – 1456 .
Silva MS , Prescott M , Campbell RE . Ontogeny and reversal of brain circuit abnormalities in a preclinical model of PCOS . JCI Insight . 2018 ; 3 ( 7 ): 99405 .
Moller DE , Flier JS . Detection of an alteration in the insulin-receptor gene in a patient with insulin resistance, acanthosis nigricans, and the polycystic ovary syndrome (type A insulin resistance) . N Engl J Med . 1988 ; 319 ( 23 ): 1526 – 1529 .
Dunaif A , Segal KR , Futterweit W , Dobrjansky A . Profound peripheral insulin resistance, independent of obesity, in polycystic ovary syndrome . Diabetes . 1989 ; 38 ( 9 ): 1165 – 1174 .
Stepto NK , Cassar S , Joham AE , Hutchison SK , Harrison CL , Goldstein RF , Teede HJ . Women with polycystic ovary syndrome have intrinsic insulin resistance on euglycaemic–hyperinsulaemic clamp . Hum Reprod . 2013 ; 28 ( 3 ): 777 – 784 .
Cree-Green M , Rahat H , Newcomer BR , Bergman BC , Brown MS , Coe GV , Newnes L , Garcia-Reyes Y , Bacon S , Thurston JE , Pyle L , Scherzinger A , Nadeau KJ . Insulin resistance, hyperinsulinemia, and mitochondria dysfunction in nonobese girls with polycystic ovarian syndrome . J Endocr Soc . 2017 ; 1 ( 7 ): 931 – 944 .
Geffner ME , Golde DW . Selective insulin action on skin, ovary, and heart in insulin-resistant states . Diabetes Care . 1988 ; 11 ( 6 ): 500 – 505 .
Wu S , Divall S , Wondisford F , Wolfe A . Reproductive tissues maintain insulin sensitivity in diet-induced obesity . Diabetes . 2012 ; 61 ( 1 ): 114 – 123 .
Rice S , Christoforidis N , Gadd C , Nikolaou D , Seyani L , Donaldson A , Margara R , Hardy K , Franks S . Impaired insulin-dependent glucose metabolism in granulosa-lutein cells from anovulatory women with polycystic ovaries . Hum Reprod . 2005 ; 20 ( 2 ): 373 – 381 .
Caprio S . Insulin: the other anabolic hormone of puberty . Acta Paediatr Suppl . 1999 ; 88 ( 433 ): 84 – 87 .
Hannon TS , Janosky J , Arslanian SA . Longitudinal study of physiologic insulin resistance and metabolic changes of puberty . Pediatr Res . 2006 ; 60 ( 6 ): 759 – 763 .
Moran LJ , Misso ML , Wild RA , Norman RJ . Impaired glucose tolerance, type 2 diabetes and metabolic syndrome in polycystic ovary syndrome: a systematic review and meta-analysis . Hum Reprod Update . 2010 ; 16 ( 4 ): 347 – 363 .
de Ferranti SD , Gauvreau K , Ludwig DS , Neufeld EJ , Newburger JW , Rifai N . Prevalence of the metabolic syndrome in American adolescents: findings from the Third National Health and Nutrition Examination Survey . Circulation . 2004 ; 110 ( 16 ): 2494 – 2497 .
Lim SS , Kakoly NS , Tan JWJ , Fitzgerald G , Bahri Khomami M , Joham AE , Cooray SD , Misso ML , Norman RJ , Harrison CL , Ranasinha S , Teede HJ , Moran LJ . Metabolic syndrome in polycystic ovary syndrome: a systematic review, meta-analysis and meta-regression . Obes Rev . 2019 ; 20 ( 2 ): 339 – 352 .
Czech MP . Insulin action and resistance in obesity and type 2 diabetes . Nat Med . 2017 ; 23 ( 7 ): 804 – 814 .
Nicol LE , O’Brien TD , Dumesic DA , Grogan T , Tarantal AF , Abbott DH . Abnormal infant islet morphology precedes insulin resistance in PCOS-like monkeys . PLoS One . 2014 ; 9 ( 9 ): e106527 .
Ramaswamy S , Grace C , Mattei AA , Siemienowicz K , Brownlee W , MacCallum J , McNeilly AS , Duncan WC , Rae MT . Developmental programming of polycystic ovary syndrome (PCOS): prenatal androgens establish pancreatic islet α/β cell ratio and subsequent insulin secretion . Sci Rep . 2016 ; 6 ( 1 ): 27408 .
Virtue S , Vidal-Puig A . Adipose tissue expandability, lipotoxicity and the metabolic syndrome—an allostatic perspective . Biochim Biophys Acta . 2010 ; 1801 ( 3 ): 338 – 349 .
Samuel VT , Shulman GI . The pathogenesis of insulin resistance: integrating signaling pathways and substrate flux . J Clin Invest . 2016 ; 126 ( 1 ): 12 – 22 .
Grundy SM . Overnutrition, ectopic lipid and the metabolic syndrome . J Investig Med . 2016 ; 64 ( 6 ): 1082 – 1086 .
Dumesic DA , Akopians AL , Madrigal VK , Ramirez E , Margolis DJ , Sarma MK , Thomas AM , Grogan TR , Haykal R , Schooler TA , Okeya BL , Abbott DH , Chazenbalk GD . Hyperandrogenism accompanies increased intra-abdominal fat storage in normal weight polycystic ovary syndrome women . J Clin Endocrinol Metab . 2016 ; 101 ( 11 ): 4178 – 4188 .
Dumesic DA , Phan JD , Leung KL , Grogan TR , Ding X , Li X , Hoyos LR , Abbott DH , Chazenbalk GD . Adipose insulin resistance in normal-weight women with polycystic ovary syndrome . J Clin Endocrinol Metab . 2019 ; 104 ( 6 ): 2171 – 2183 .
Ibáñez L , Del Río L , Díaz M , Sebastiani G , Pozo ÓJ , López-Bermejo A , de Zegher F . Normalizing ovulation rate by preferential reduction of hepato-visceral fat in adolescent girls with polycystic ovary syndrome . J Adolesc Health . 2017 ; 61 ( 4 ): 446 – 453 .
Trottier A , Battista MC , Geller DH , Moreau B , Carpentier AC , Simoneau-Roy J , Baillargeon JP . Adipose tissue insulin resistance in peripubertal girls with first-degree family history of polycystic ovary syndrome . Fertil Steril . 2012 ; 98 ( 6 ): 1627 – 1634 .
Torchen LC , Fogel NR , Brickman WJ , Paparodis R , Dunaif A . Persistent apparent pancreatic β-cell defects in premenarchal PCOS relatives . J Clin Endocrinol Metab . 2014 ; 99 ( 10 ): 3855 – 3862 .
Osmond C , Barker DJ . Fetal, infant, and childhood growth are predictors of coronary heart disease, diabetes, and hypertension in adult men and women . Environ Health Perspect . 2000 ; 108 ( Suppl 3 ): 545 – 553 .
Koivuaho E , Laru J , Ojaniemi M , Puukka K , Kettunen J , Tapanainen JS , Franks S , Järvelin MR , Morin-Papunen L , Sebert S , Piltonen TT . Age at adiposity rebound in childhood is associated with PCOS diagnosis and obesity in adulthood-longitudinal analysis of BMI data from birth to age 46 in cases of PCOS [published online ahead of print 4 February 2019] . Int J Obes (Lond) . 2019 ; 43 ( 7 ): 1370 – 1379 .
Ibáñez L , Ong KK , López-Bermejo A , Dunger DB , de Zegher F . Hyperinsulinaemic androgen excess in adolescent girls [published correction appears in Nat Rev Endocrinol. 2014; 10 :578] . Nat Rev Endocrinol . 2014 ; 10 ( 8 ): 499 – 508 .
de Zegher F , López-Bermejo A , Ibáñez L . Central obesity, faster maturation, and “PCOS” in girls . Trends Endocrinol Metab . 2018 ; 29 ( 12 ): 815 – 818 .
de Zegher F , Reinehr T , Malpique R , Darendeliler F , López-Bermejo A , Ibáñez L . Reduced prenatal weight gain and/or augmented postnatal weight gain precedes polycystic ovary syndrome in adolescent girls . Obesity (Silver Spring) . 2017 ; 25 ( 9 ): 1486 – 1489 .
O’Reilly MW , Kempegowda P , Walsh M , Taylor AE , Manolopoulos KN , Allwood JW , Semple RK , Hebenstreit D , Dunn WB , Tomlinson JW , Arlt W . AKR1C3-mediated adipose androgen generation drives lipotoxicity in women with polycystic ovary syndrome . J Clin Endocrinol Metab . 2017 ; 102 ( 9 ): 3327 – 3339 .
O’Reilly MW , House PJ , Tomlinson JW . Understanding androgen action in adipose tissue . J Steroid Biochem Mol Biol . 2014 ; 143 : 277 – 284 .
Kim JY , Tfayli H , Michaliszyn SF , Arslanian S . Impaired lipolysis, diminished fat oxidation, and metabolic inflexibility in obese girls with polycystic ovary syndrome . J Clin Endocrinol Metab . 2018 ; 103 ( 2 ): 546 – 554 .
Varlamov O , Bishop CV , Handu M , Takahashi D , Srinivasan S , White A , Roberts CT Jr . Combined androgen excess and Western-style diet accelerates adipose tissue dysfunction in young adult, female nonhuman primates . Hum Reprod . 2017 ; 32 ( 9 ): 1892 – 1902 .
Filippou P , Homburg R . Is foetal hyperexposure to androgens a cause of PCOS ? Hum Reprod Update . 2017 ; 23 ( 4 ): 421 – 432 .
Abbott DH , Dumesic DA , Franks S . Developmental origin of polycystic ovary syndrome—a hypothesis . J Endocrinol . 2002 ; 174 ( 1 ): 1 – 5 .
Abbott DH , Barnett DK , Bruns CM , Dumesic DA . Androgen excess fetal programming of female reproduction: a developmental aetiology for polycystic ovary syndrome ? Hum Reprod Update . 2005 ; 11 ( 4 ): 357 – 374 .
Witchel SF . Congenital adrenal hyperplasia . J Pediatr Adolesc Gynecol . 2017 ; 30 ( 5 ): 520 – 534 .
Abbott DH , Bacha F . Ontogeny of polycystic ovary syndrome and insulin resistance in utero and early childhood . Fertil Steril . 2013 ; 100 ( 1 ): 2 – 11 .
Barrett ES , Hoeger KM , Sathyanarayana S , Abbott DH , Redmon JB , Nguyen RHN , Swan SH . Anogenital distance in newborn daughters of women with polycystic ovary syndrome indicates fetal testosterone exposure . J Dev Orig Health Dis . 2018 ; 9 ( 3 ): 307 – 314 .
Keller E , Chazenbalk GD , Aguilera P , Madrigal V , Grogan T , Elashoff D , Dumesic DA , Abbott DH . Impaired preadipocyte differentiation into adipocytes in subcutaneous abdominal adipose of PCOS-like female rhesus monkeys . Endocrinology . 2014 ; 155 ( 7 ): 2696 – 2703 .
True C , Abbott DH , Roberts CT Jr , Varlamov O . Sex differences in androgen regulation of metabolism in nonhuman primates . Adv Exp Med Biol . 2017 ; 1043 : 559 – 574 .
Sharpton SR , Ajmera V , Loomba R . Emerging role of the gut microbiome in nonalcoholic fatty liver disease: from composition to function . Clin Gastroenterol Hepatol . 2019 ; 17 ( 2 ): 296 – 306 .
Pedersen HK , Gudmundsdottir V , Nielsen HB , Hyotylainen T , Nielsen T , Jensen BA , Forslund K , Hildebrand F , Prifti E , Falony G , Le Chatelier E , Levenez F , Doré J , Mattila I , Plichta DR , Pöhö P , Hellgren LI , Arumugam M , Sunagawa S , Vieira-Silva S , Jørgensen T , Holm JB , Trošt K , Kristiansen K , Brix S , Raes J , Wang J , Hansen T , Bork P , Brunak S , Oresic M , Ehrlich SD , Pedersen O ; MetaHIT Consortium . Human gut microbes impact host serum metabolome and insulin sensitivity . Nature . 2016 ; 535 ( 7612 ): 376 – 381 .
Insenser M , Murri M , Del Campo R , Martínez-García MÁ , Fernández-Durán E , Escobar-Morreale HF . Gut microbiota and the polycystic ovary syndrome: influence of sex, sex hormones, and obesity . J Clin Endocrinol Metab . 2018 ; 103 ( 7 ): 2552 – 2562 .
Torres PJ , Siakowska M , Banaszewska B , Pawelczyk L , Duleba AJ , Kelley ST , Thackray VG . Gut microbial diversity in women with polycystic ovary syndrome correlates with hyperandrogenism . J Clin Endocrinol Metab . 2018 ; 103 ( 4 ): 1502 – 1511 .
Thackray VG . Sex, microbes, and polycystic ovary syndrome . Trends Endocrinol Metab . 2019 ; 30 ( 1 ): 54 – 65 .
Vink JM , Sadrzadeh S , Lambalk CB , Boomsma DI . Heritability of polycystic ovary syndrome in a Dutch twin-family study . J Clin Endocrinol Metab . 2006 ; 91 ( 6 ): 2100 – 2104 .
Chen ZJ , Zhao H , He L , Shi Y , Qin Y , Shi Y , Li Z , You L , Zhao J , Liu J , Liang X , Zhao X , Zhao J , Sun Y , Zhang B , Jiang H , Zhao D , Bian Y , Gao X , Geng L , Li Y , Zhu D , Sun X , Xu JE , Hao C , Ren CE , Zhang Y , Chen S , Zhang W , Yang A , Yan J , Li Y , Ma J , Zhao Y . Genome-wide association study identifies susceptibility loci for polycystic ovary syndrome on chromosome 2p16.3, 2p21 and 9q33.3 . Nat Genet . 2011 ; 43 ( 1 ): 55 – 59 .
Shi Y , Zhao H , Shi Y , Cao Y , Yang D , Li Z , Zhang B , Liang X , Li T , Chen J , Shen J , Zhao J , You L , Gao X , Zhu D , Zhao X , Yan Y , Qin Y , Li W , Yan J , Wang Q , Zhao J , Geng L , Ma J , Zhao Y , He G , Zhang A , Zou S , Yang A , Liu J , Li W , Li B , Wan C , Qin Y , Shi J , Yang J , Jiang H , Xu JE , Qi X , Sun Y , Zhang Y , Hao C , Ju X , Zhao D , Ren CE , Li X , Zhang W , Zhang Y , Zhang J , Wu D , Zhang C , He L , Chen ZJ . Genome-wide association study identifies eight new risk loci for polycystic ovary syndrome . Nat Genet . 2012 ; 44 ( 9 ): 1020 – 1025 .
Hayes MG , Urbanek M , Ehrmann DA , Armstrong LL , Lee JY , Sisk R , Karaderi T , Barber TM , McCarthy MI , Franks S , Lindgren CM , Welt CK , Diamanti-Kandarakis E , Panidis D , Goodarzi MO , Azziz R , Zhang Y , James RG , Olivier M , Kissebah AH , Stener-Victorin E , Legro RS , Dunaif A ; Reproductive Medicine Network . Genome-wide association of polycystic ovary syndrome implicates alterations in gonadotropin secretion in European ancestry populations [published correction appears in Nat Commun . 2016; 7 :10762] . Nat Commun . 2015 ; 6 ( 1 ): 7502 .
Day FR , Hinds DA , Tung JY , Stolk L , Styrkarsdottir U , Saxena R , Bjonnes A , Broer L , Dunger DB , Halldorsson BV , Lawlor DA , Laval G , Mathieson I , McCardle WL , Louwers Y , Meun C , Ring S , Scott RA , Sulem P , Uitterlinden AG , Wareham NJ , Thorsteinsdottir U , Welt C , Stefansson K , Laven JS , Ong KK , Perry JR . Causal mechanisms and balancing selection inferred from genetic associations with polycystic ovary syndrome . Nat Commun . 2015 ; 6 ( 1 ): 8464 .
Fessler DM , Natterson-Horowitz B , Azziz R . Evolutionary determinants of polycystic ovary syndrome: part 2 . Fertil Steril . 2016 ; 106 ( 1 ): 42 – 47 .
Day F , Karaderi T , Jones MR , Meun C , He C , Drong A , Kraft P , Lin N , Huang H , Broer L , Magi R , Saxena R , Laisk T , Urbanek M , Hayes MG , Thorleifsson G , Fernandez-Tajes J , Mahajan A , Mullin BH , Stuckey BGA , Spector TD , Wilson SG , Goodarzi MO , Davis L , Obermayer-Pietsch B , Uitterlinden AG , Anttila V , Neale BM , Jarvelin MR , Fauser B , Kowalska I , Visser JA , Andersen M , Ong K , Stener-Victorin E , Ehrmann D , Legro RS , Salumets A , McCarthy MI , Morin-Papunen L , Thorsteinsdottir U , Stefansson K , Styrkarsdottir U , Perry JRB , Dunaif A , Laven J , Franks S , Lindgren CM , Welt CK ; 23andMe Research Team . Large-scale genome-wide meta-analysis of polycystic ovary syndrome suggests shared genetic architecture for different diagnosis criteria . PLoS Genet . 2018 ; 14 ( 12 ): e1007813 .
Dapas M , Sisk R , Legro RS , Urbanek M , Dunaif A , Hayes MG . Family-based quantitative trait meta-analysis implicates rare noncoding variants in DENND1A in polycystic ovary syndrome [published online ahead of print 30 April 2019]. J Clin Endocrinol Metab. doi: 10.1210/jc.2018-02496 .
Kokosar M , Benrick A , Perfilyev A , Fornes R , Nilsson E , Maliqueo M , Behre CJ , Sazonova A , Ohlsson C , Ling C , Stener-Victorin E . Epigenetic and transcriptional alterations in human adipose tissue of polycystic ovary syndrome [published correction appears in Sci Rep . 2016; 6 :25321] . Sci Rep . 2016 ; 6 ( 1 ): 22883 .
Nilsson E , Benrick A , Kokosar M , Krook A , Lindgren E , Källman T , Martis MM , Højlund K , Ling C , Stener-Victorin E . Transcriptional and epigenetic changes influencing skeletal muscle metabolism in women with polycystic ovary syndrome . J Clin Endocrinol Metab . 2018 ; 103 ( 12 ): 4465 – 4477 .
Dumesic DA , Oberfield SE , Stener-Victorin E , Marshall JC , Laven JS , Legro RS . Scientific statement on the diagnostic criteria, epidemiology, pathophysiology, and molecular genetics of polycystic ovary syndrome . Endocr Rev . 2015 ; 36 ( 5 ): 487 – 525 .
Johnson T , Kaplan L , Ouyang P , Rizza P . National Institutes of Health Evidence-Based Methodology Workshop on Polycystic Ovary Syndrome. NIH EbMW Reports. Bethesda, MD: National Institutes of Health, 2012;1:1–14. Available at: https://prevention.nih.gov/sites/default/files/2018-06/FinalReport.pdf . Accessed 17 February 2019 .
Lizneva D , Suturina L , Walker W , Brakta S , Gavrilova-Jordan L , Azziz R . Criteria, prevalence, and phenotypes of polycystic ovary syndrome . Fertil Steril . 2016 ; 106 ( 1 ): 6 – 15 .
Teede HJ , Misso ML , Costello MF , Dokras A , Laven J , Moran L , Piltonen T , Norman RJ ; International PCOS Network . Recommendations from the international evidence-based guideline for the assessment and management of polycystic ovary syndrome . Clin Endocrinol (Oxf) . 2018 ; 89 ( 3 ): 251 – 268 .
Ibáñez L , Oberfield SE , Witchel S , Auchus RJ , Chang RJ , Codner E , Dabadghao P , Darendeliler F , Elbarbary NS , Gambineri A , Garcia Rudaz C , Hoeger KM , López-Bermejo A , Ong K , Peña AS , Reinehr T , Santoro N , Tena-Sempere M , Tao R , Yildiz BO , Alkhayyat H , Deeb A , Joel D , Horikawa R , de Zegher F , Lee PA . An international consortium update: pathophysiology, diagnosis, and treatment of polycystic ovarian syndrome in adolescence . Horm Res Paediatr . 2017 ; 88 ( 6 ): 371 – 395 .
Witchel SF , Oberfield S , Rosenfield RL , Codner E , Bonny A , Ibáñez L , Pena A , Horikawa R , Gomez-Lobo V , Joel D , Tfayli H , Arslanian S , Dabadghao P , Garcia Rudaz C , Lee PA . The diagnosis of polycystic ovary syndrome during adolescence . Horm Res Paediatr . 2015 ; 83 ( 6 ): 376 – 389 .
Trapp CM , Oberfield SE . Recommendations for treatment of nonclassic congenital adrenal hyperplasia (NCCAH): an update . Steroids . 2012 ; 77 ( 4 ): 342 – 346 .
Pall M , Azziz R , Beires J , Pignatelli D . The phenotype of hirsute women: a comparison of polycystic ovary syndrome and 21-hydroxylase-deficient nonclassic adrenal hyperplasia . Fertil Steril . 2010 ; 94 ( 2 ): 684 – 689 .
Biro FM , Pajak A , Wolff MS , Pinney SM , Windham GC , Galvez MP , Greenspan LC , Kushi LH , Teitelbaum SL . Age of menarche in a longitudinal US cohort . J Pediatr Adolesc Gynecol . 2018 ; 31 ( 4 ): 339 – 345 .
Chumlea WC , Schubert CM , Roche AF , Kulin HE , Lee PA , Himes JH , Sun SS . Age at menarche and racial comparisons in US girls . Pediatrics . 2003 ; 111 ( 1 ): 110 – 113 .
Legro RS , Lin HM , Demers LM , Lloyd T . Rapid maturation of the reproductive axis during perimenarche independent of body composition . J Clin Endocrinol Metab . 2000 ; 85 ( 3 ): 1021 – 1025 .
Vihko R , Apter D . Endocrine characteristics of adolescent menstrual cycles: impact of early menarche . J Steroid Biochem . 1984 ; 20 ( 1 ): 231 – 236 .
Peña AS , Doherty DA , Atkinson HC , Hickey M , Norman RJ , Hart R . The majority of irregular menstrual cycles in adolescence are ovulatory: results of a prospective study . Arch Dis Child . 2018 ; 103 ( 3 ): 235 – 239 .
Gunn HM , Tsai MC , McRae A , Steinbeck KS . Menstrual patterns in the first gynecological year: a systematic review . J Pediatr Adolesc Gynecol . 2018 ; 31 ( 6 ): 557 – 565.e6 .
Sun BZ , Kangarloo T , Adams JM , Sluss PM , Welt CK , Chandler DW , Zava DT , McGrath JA , Umbach DM , Hall JE , Shaw ND . Healthy post-menarchal adolescent girls demonstrate multi-level reproductive axis immaturity . J Clin Endocrinol Metab . 2019 ; 104 ( 2 ): 613 – 623 .
Treloar AE , Boynton RE , Behn BG , Brown BW . Variation of the human menstrual cycle through reproductive life . Int J Fertil . 1967 ; 12 ( 1 Pt 2 ): 77 – 126 .
van Hooff MH , Voorhorst FJ , Kaptein MB , Hirasing RA , Koppenaal C , Schoemaker J . Predictive value of menstrual cycle pattern, body mass index, hormone levels and polycystic ovaries at age 15 years for oligo-amenorrhoea at age 18 years . Hum Reprod . 2004 ; 19 ( 2 ): 383 – 392 .
Southam AL , Richart RM . The prognosis for adolescents with menstrual abnormalities . Am J Obstet Gynecol . 1966 ; 94 ( 5 ): 637 – 645 .
Ferriman D , Gallwey JD . Clinical assessment of body hair growth in women . J Clin Endocrinol Metab . 1961 ; 21 ( 11 ): 1440 – 1447 .
Yildiz BO , Bolour S , Woods K , Moore A , Azziz R . Visually scoring hirsutism . Hum Reprod Update . 2010 ; 16 ( 1 ): 51 – 64 .
Rosner W , Vesper H ; Endocrine Society ; American Association for Clinical Chemistry ; American Association of Clinical Endocrinologists ; Androgen Excess/PCOS Society ; American Society for Bone and Mineral Research ; American Society for Reproductive Medicine ; American Urological Association ; Association of Public Health Laboratories ; Endocrine Society ; Laboratory Corporation of America ; North American Menopause Society ; Pediatric Endocrine Society . Toward excellence in testosterone testing: a consensus statement . J Clin Endocrinol Metab . 2010 ; 95 ( 10 ): 4542 – 4548 .
Kyriakopoulou L , Yazdanpanah M , Colantonio DA , Chan MK , Daly CH , Adeli K . A sensitive and rapid mass spectrometric method for the simultaneous measurement of eight steroid hormones and CALIPER pediatric reference intervals . Clin Biochem . 2013 ; 46 ( 7-8 ): 642 – 651 .
Turcu AF , Auchus RJ . Clinical significance of 11-oxygenated androgens . Curr Opin Endocrinol Diabetes Obes . 2017 ; 24 ( 3 ): 252 – 259 .
Pretorius E , Arlt W , Storbeck KH . A new dawn for androgens: novel lessons from 11-oxygenated C19 steroids . Mol Cell Endocrinol . 2017 ; 441 : 76 – 85 .
Dewailly D , Lujan ME , Carmina E , Cedars MI , Laven J , Norman RJ , Escobar-Morreale HF . Definition and significance of polycystic ovarian morphology: a task force report from the Androgen Excess and Polycystic Ovary Syndrome Society . Hum Reprod Update . 2014 ; 20 ( 3 ): 334 – 352 .
Codner E , Villarroel C , Eyzaguirre FC , López P , Merino PM , Pérez-Bravo F , Iñiguez G , Cassorla F . Polycystic ovarian morphology in postmenarchal adolescents . Fertil Steril . 2011 ; 95 ( 2 ): 702 – 706.e1–2 .
Armengaud JB , Charkaluk ML , Trivin C , Tardy V , Bréart G , Brauner R , Chalumeau M . Precocious pubarche: distinguishing late-onset congenital adrenal hyperplasia from premature adrenarche . J Clin Endocrinol Metab . 2009 ; 94 ( 8 ): 2835 – 2840 .
Pigny P , Merlen E , Robert Y , Cortet-Rudelli C , Decanter C , Jonard S , Dewailly D . Elevated serum level of anti-Mullerian hormone in patients with polycystic ovary syndrome: relationship to the ovarian follicle excess and to the follicular arrest . J Clin Endocrinol Metab . 2003 ; 88 ( 12 ): 5957 – 5962 .
Tokmak A , Timur H , Aksoy RT , Çınar M , Yılmaz N . Is anti-Mullerian hormone a good diagnostic marker for adolescent and young adult patients with Polycystic ovary syndrome ? Turk J Obstet Gynecol . 2015 ; 12 ( 4 ): 199 – 204 .
Sopher AB , Grigoriev G , Laura D , Cameo T , Lerner JP , Chang RJ , McMahon DJ , Oberfield SE . Anti-Mullerian hormone may be a useful adjunct in the diagnosis of polycystic ovary syndrome in nonobese adolescents . J Pediatr Endocrinol Metab . 2014 ; 27 ( 11–12 ): 1175 – 1179 .
Kim JY , Tfayli H , Michaliszyn SF , Lee S , Nasr A , Arslanian S . Anti-Müllerian hormone in obese adolescent girls with polycystic ovary syndrome . J Adolesc Health . 2017 ; 60 ( 3 ): 333 – 339 .
Sultan C , Paris F . Clinical expression of polycystic ovary syndrome in adolescent girls . Fertil Steril . 2006 ; 86 ( Suppl 1 ): S6 .
Gibson-Helm M , Teede H , Dunaif A , Dokras A . Delayed diagnosis and a lack of information associated with dissatisfaction in women with polycystic ovary syndrome . J Clin Endocrinol Metab . 2017 ; 102 ( 2 ): 604 – 612 .
Cree-Green M . Worldwide dissatisfaction with the diagnostic process and initial treatment of PCOS . J Clin Endocrinol Metab . 2017 ; 102 ( 2 ): 375 – 378 .
Trent ME , Rich M , Austin SB , Gordon CM . Fertility concerns and sexual behavior in adolescent girls with polycystic ovary syndrome: implications for quality of life . J Pediatr Adolesc Gynecol . 2003 ; 16 ( 1 ): 33 – 37 .
Lim SS , Davies MJ , Norman RJ , Moran LJ . Overweight, obesity and central obesity in women with polycystic ovary syndrome: a systematic review and meta-analysis . Hum Reprod Update . 2012 ; 18 ( 6 ): 618 – 637 .
Ells LJ , Rees K , Brown T , Mead E , Al-Khudairy L , Azevedo L , McGeechan GJ , Baur L , Loveman E , Clements H , Rayco-Solon P , Farpour-Lambert N , Demaio A . Interventions for treating children and adolescents with overweight and obesity: an overview of Cochrane reviews . Int J Obes . 2018 ; 42 ( 11 ): 1823 – 1833 .
Jakubowski KP , Black JJ , El Nokali NE , Belendiuk KA , Hannon TS , Arslanian SA , Rofey DL . Parents’ readiness to change affects BMI reduction outcomes in adolescents with polycystic ovary syndrome . j obes . 2012 ; 2012 : 298067 .
Lass N , Kleber M , Winkel K , Wunsch R , Reinehr T . Effect of lifestyle intervention on features of polycystic ovarian syndrome, metabolic syndrome, and intima-media thickness in obese adolescent girls . J Clin Endocrinol Metab . 2011 ; 96 ( 11 ): 3533 – 3540 .
Kaczmarek C , Haller DM , Yaron M . Health-related quality of life in adolescents and young adults with polycystic ovary syndrome: a systematic review . J Pediatr Adolesc Gynecol . 2016 ; 29 ( 6 ): 551 – 557 .
Moran LJ , Hutchison SK , Norman RJ , Teede HJ . Lifestyle changes in women with polycystic ovary syndrome . Cochrane Database Syst Rev . 2011 ; ( 7 ): CD007506 .
Domecq JP , Prutsky G , Mullan RJ , Hazem A , Sundaresh V , Elamin MB , Phung OJ , Wang A , Hoeger K , Pasquali R , Erwin P , Bodde A , Montori VM , Murad MH . Lifestyle modification programs in polycystic ovary syndrome: systematic review and meta-analysis . J Clin Endocrinol Metab . 2013 ; 98 ( 12 ): 4655 – 4663 .
Hoeger K , Davidson K , Kochman L , Cherry T , Kopin L , Guzick DS . The impact of metformin, oral contraceptives, and lifestyle modification on polycystic ovary syndrome in obese adolescent women in two randomized, placebo-controlled clinical trials . J Clin Endocrinol Metab . 2008 ; 93 ( 11 ): 4299 – 4306 .
Harris-Glocker M , Davidson K , Kochman L , Guzick D , Hoeger K . Improvement in quality-of-life questionnaire measures in obese adolescent females with polycystic ovary syndrome treated with lifestyle changes and oral contraceptives, with or without metformin . Fertil Steril . 2010 ; 93 ( 3 ): 1016 – 1019 .
Ornstein RM , Copperman NM , Jacobson MS . Effect of weight loss on menstrual function in adolescents with polycystic ovary syndrome . J Pediatr Adolesc Gynecol . 2011 ; 24 ( 3 ): 161 – 165 .
Marzouk TM , Sayed Ahmed WA . Effect of dietary weight loss on menstrual regularity in obese young adult women with polycystic ovary syndrome . J Pediatr Adolesc Gynecol . 2015 ; 28 ( 6 ): 457 – 461 .
Wong JM , Gallagher M , Gooding H , Feldman HA , Gordon CM , Ludwig DS , Ebbeling CB . A randomized pilot study of dietary treatments for polycystic ovary syndrome in adolescents . Pediatr Obes . 2016 ; 11 ( 3 ): 210 – 220 .
Poitras VJ , Gray CE , Borghese MM , Carson V , Chaput JP , Janssen I , Katzmarzyk PT , Pate RR , Connor Gorber S , Kho ME , Sampson M , Tremblay MS . Systematic review of the relationships between objectively measured physical activity and health indicators in school-aged children and youth . Appl Physiol Nutr Metab . 2016 ; 41 ( 6 Suppl 3 ): S197 – S239 .
Harrison CL , Lombard CB , Moran LJ , Teede HJ . Exercise therapy in polycystic ovary syndrome: a systematic review . Hum Reprod Update . 2011 ; 17 ( 2 ): 171 – 183 .
Benham JL , Yamamoto JM , Friedenreich CM , Rabi DM , Sigal RJ . Role of exercise training in polycystic ovary syndrome: a systematic review and meta-analysis . Clin Obes . 2018 ; 8 ( 4 ): 275 – 284 .
Nidhi R , Padmalatha V , Nagarathna R , Amritanshu R . Effect of holistic yoga program on anxiety symptoms in adolescent girls with polycystic ovarian syndrome: a randomized control trial . Int J Yoga . 2012 ; 5 ( 2 ): 112 – 117 .
Carson V , Hunter S , Kuzik N , Gray CE , Poitras VJ , Chaput JP , Saunders TJ , Katzmarzyk PT , Okely AD , Connor Gorber S , Kho ME , Sampson M , Lee H , Tremblay MS . Systematic review of sedentary behaviour and health indicators in school-aged children and youth: an update . Appl Physiol Nutr Metab . 2016 ; 41 ( 6 Suppl 3 ): S240 – S265 .
Rofey DL , Szigethy EM , Noll RB , Dahl RE , Lobst E , Arslanian SA . Cognitive–behavioral therapy for physical and emotional disturbances in adolescents with polycystic ovary syndrome: a pilot study . J Pediatr Psychol . 2009 ; 34 ( 2 ): 156 – 163 .
Li L , Feng Q , Ye M , He Y , Yao A , Shi K . Metabolic effect of obesity on polycystic ovary syndrome in adolescents: a meta-analysis . J Obstet Gynaecol . 2017 ; 37 ( 8 ): 1036 – 1047 .
Trent M , Austin SB , Rich M , Gordon CM . Overweight status of adolescent girls with polycystic ovary syndrome: body mass index as mediator of quality of life . Ambul Pediatr . 2005 ; 5 ( 2 ): 107 – 111 .
Wang T , McNeill AM , Chen Y , Senderak M , Shankar RR . Metformin prescription patterns among US adolescents aged 10–19 years: 2009–2013 . J Clin Pharm Ther . 2016 ; 41 ( 2 ): 229 – 236 .
Ladson G , Dodson WC , Sweet SD , Archibong AE , Kunselman AR , Demers LM , Lee PA , Williams NI , Coney P , Legro RS . Effects of metformin in adolescents with polycystic ovary syndrome undertaking lifestyle therapy: a pilot randomized double-blind study . Fertil Steril . 2011 Jun 30; 95 ( 8 ): 2595 – 2598 .e1–6.
Naderpoor N , Shorakae S , de Courten B , Misso ML , Moran LJ , Teede HJ . Metformin and lifestyle modification in polycystic ovary syndrome: systematic review and meta-analysis . Hum Reprod Update . 2015 ; 21 ( 5 ): 560 – 574 .
Allen HF , Mazzoni C , Heptulla RA , Murray MA , Miller N , Koenigs L , Reiter EO . Randomized controlled trial evaluating response to metformin versus standard therapy in the treatment of adolescents with polycystic ovary syndrome . J Pediatr Endocrinol Metab . 2005 ; 18 ( 8 ): 761 – 768 .
Bridger T , MacDonald S , Baltzer F , Rodd C . Randomized placebo-controlled trial of metformin for adolescents with polycystic ovary syndrome . Arch Pediatr Adolesc Med . 2006 ; 160 ( 3 ): 241 – 246 .
Al-Zubeidi H , Klein KO . Randomized clinical trial evaluating metformin versus oral contraceptive pills in the treatment of adolescents with polycystic ovarian syndrome . J Pediatr Endocrinol Metab . 2015 ; 28 ( 7-8 ): 853 – 858 .
El Maghraby HA , Nafee T , Guiziry D , Elnashar A . Randomized controlled trial of the effects of metformin versus combined oral contraceptives in adolescent PCOS women through a 24 month follow up period . Middle East Fertil Soc J . 2015 ; 20 ( 3 ): 131 – 137 .
Al Khalifah RA , Florez ID , Dennis B , Thabane L , Bassilious E . Metformin or oral contraceptives for adolescents with polycystic ovarian syndrome: a meta-analysis . Pediatrics . 2016 ; 137 ( 5 ): e20154089 .
Cosma M , Swiglo BA , Flynn DN , Kurtz DM , Labella ML , Mullan RJ , Elamin MB , Erwin PJ , Montori VM . Clinical review: insulin sensitizers for the treatment of hirsutism: a systematic review and metaanalyses of randomized controlled trials . J Clin Endocrinol Metab . 2008 ; 93 ( 4 ): 1135 – 1142 .
Barrionuevo P , Nabhan M , Altayar O , Wang Z , Erwin PJ , Asi N , Martin KA , Murad MH . Treatment options for hirsutism: a systematic review and network meta-analysis . J Clin Endocrinol Metab . 2018 ; 103 ( 4 ): 1258 – 1264 .
Bird ST , Hartzema AG , Brophy JM , Etminan M , Delaney JA . Risk of venous thromboembolism in women with polycystic ovary syndrome: a population-based matched cohort analysis . CMAJ . 2013 ; 185 ( 2 ): E115 – E120 .
Ozdemir S , Görkemli H , Gezginç K , Ozdemir M , Kiyici A . Clinical and metabolic effects of medroxyprogesterone acetate and ethinyl estradiol plus drospirenone in women with polycystic ovary syndrome . Int J Gynaecol Obstet . 2008 ; 103 ( 1 ): 44 – 49 .
Chung JP , Yiu AK , Chung TK , Chan SS . A randomized crossover study of medroxyprogesterone acetate and Diane-35 in adolescent girls with polycystic ovarian syndrome . J Pediatr Adolesc Gynecol . 2014 ; 27 ( 3 ): 166 – 171 .
Pasquali R , Gambineri A . Therapy in endocrine disease: treatment of hirsutism in the polycystic ovary syndrome . Eur J Endocrinol . 2013 ; 170 ( 2 ): R75 – R90 .
Somani N , Turvy D . Hirsutism: an evidence-based treatment update . Am J Clin Dermatol . 2014 ; 15 ( 3 ): 247 – 266 .
van Zuuren EJ , Fedorowicz Z . Interventions for hirsutism excluding laser and photoepilation therapy alone: abridged Cochrane systematic review including GRADE assessments . Br J Dermatol . 2016 ; 175 ( 1 ): 45 – 61 .
Wagner RF Jr , Tomich JM , Grande DJ . Electrolysis and thermolysis for permanent hair removal . J Am Acad Dermatol . 1985 ; 12 ( 3 ): 441 – 449 .
Jackson J , Caro JJ , Caro G , Garfield F , Huber F , Zhou W , Lin CS , Shander D , Schrode K ; Eflornithine HCl Study Group . The effect of eflornithine 13.9% cream on the bother and discomfort due to hirsutism . Int J Dermatol . 2007 ; 46 ( 9 ): 976 – 981 .
Wolf JE Jr , Shander D , Huber F , Jackson J , Lin CS , Mathes BM , Schrode K ; Eflornithine HCl Study Group . Randomized, double-blind clinical evaluation of the efficacy and safety of topical eflornithine HCl 13.9% cream in the treatment of women with facial hair . Int J Dermatol . 2007 ; 46 ( 1 ): 94 – 98 .
Gan SD , Graber EM . Laser hair removal: a review . Dermatol Surg . 2013 ; 39 ( 6 ): 823 – 838 .
Haedersdal M , Gøtzsche PC . Laser and photoepilation for unwanted hair growth . Cochrane Database Syst Rev . 2006 ; ( 4 ): CD004684 .
Maziar A , Farsi N , Mandegarfard M , Babakoohi S , Gorouhi F , Dowlati Y , Firooz A . Unwanted facial hair removal with laser treatment improves quality of life of patients . J Cosmet Laser Ther . 2010 ; 12 ( 1 ): 7 – 9 .
Clayton WJ , Lipton M , Elford J , Rustin M , Sherr L . A randomized controlled trial of laser treatment among hirsute women with polycystic ovary syndrome . Br J Dermatol . 2005 ; 152 ( 5 ): 986 – 992 .
Town G , Ash C , Dierickx C , Fritz K , Bjerring P , Haedersdal M . Guidelines on the safety of light-based home-use hair removal devices from the European Society for Laser Dermatology . J Eur Acad Dermatol Venereol . 2012 ; 26 ( 7 ): 799 – 811 .
Town G , Botchkareva NV , Uzunbajakava NE , Nuijs T , van Vlimmeren M , Ash C , Dierickx C . Light-based home-use devices for hair removal: why do they work and how effective they are? [published online ahead of print 25 January 2019] . Lasers Surg Med . 2019 ; 51( 6 ):481-490 .
Thaysen-Petersen D , Bjerring P , Dierickx C , Nash JF , Town G , Haedersdal M . A systematic review of light-based home-use devices for hair removal and considerations on human safety . J Eur Acad Dermatol Venereol . 2012 ; 26 ( 5 ): 545 – 553 .
Creatsas G , Hassan E , Deligeoroglou E , Tolis G , Aravantinos D . Treatment of polycystic ovarian disease during adolescence with ethinylestradiol/cyproterone acetate versus a D-Tr-6-LHRH analog . Int J Gynaecol Obstet . 1993 ; 42 ( 2 ): 147 – 153 .
Mastorakos G , Koliopoulos C , Creatsas G . Androgen and lipid profiles in adolescents with polycystic ovary syndrome who were treated with two forms of combined oral contraceptives . Fertil Steril . 2002 ; 77 ( 5 ): 919 – 927 .
Martin KA , Chang RJ , Ehrmann DA , Ibanez L , Lobo RA , Rosenfield RL , Shapiro J , Montori VM , Swiglo BA . Evaluation and treatment of hirsutism in premenopausal women: an Endocrine Society clinical practice guideline . J Clin Endocrinol Metab . 2008 ; 93 ( 4 ): 1105 – 1120 .
Ibáñez L , Ong K , Ferrer A , Amin R , Dunger D , de Zegher F . Low-dose flutamide-metformin therapy reverses insulin resistance and reduces fat mass in nonobese adolescents with ovarian hyperandrogenism . J Clin Endocrinol Metab . 2003 ; 88 ( 6 ): 2600 – 2606 .
Domecq JP , Prutsky G , Mullan RJ , Sundaresh V , Wang AT , Erwin PJ , Welt C , Ehrmann D , Montori VM , Murad MH . Adverse effects of the common treatments for polycystic ovary syndrome: a systematic review and meta-analysis . J Clin Endocrinol Metab . 2013 ; 98 ( 12 ): 4646 – 4654 .
Eichenfield LF , Krakowski AC , Piggott C , Del Rosso J , Baldwin H , Friedlander SF , Levy M , Lucky A , Mancini AJ , Orlow SJ , Yan AC , Vaux KK , Webster G , Zaenglein AL , Thiboutot DM ; American Acne and Rosacea Society . Evidence-based recommendations for the diagnosis and treatment of pediatric acne . Pediatrics . 2013 ; 131 ( Suppl 3 ): S163 – S186 .
Mwanthi M , Zaenglein AL . Update in the management of acne in adolescence . Curr Opin Pediatr . 2018 ; 30 ( 4 ): 492 – 498 .
Palmert MR , Gordon CM , Kartashov AI , Legro RS , Emans SJ , Dunaif A . Screening for abnormal glucose tolerance in adolescents with polycystic ovary syndrome . J Clin Endocrinol Metab . 2002 ; 87 ( 3 ): 1017 – 1023 .
Kakoly NS , Khomami MB , Joham AE , Cooray SD , Misso ML , Norman RJ , Harrison CL , Ranasinha S , Teede HJ , Moran LJ . Ethnicity, obesity and the prevalence of impaired glucose tolerance and type 2 diabetes in PCOS: a systematic review and meta-regression . Hum Reprod Update . 2018 ; 24 ( 4 ): 455 – 467 .
Trent ME , Rich M , Austin SB , Gordon CM . Quality of life in adolescent girls with polycystic ovary syndrome . Arch Pediatr Adolesc Med . 2002 ; 156 ( 6 ): 556 – 560 .
Jones GL , Hall JM , Lashen HL , Balen AH , Ledger WL . Health-related quality of life among adolescents with polycystic ovary syndrome . J Obstet Gynecol Neonatal Nurs . 2011 ; 40 ( 5 ): 577 – 588 .
Guidi J , Gambineri A , Zanotti L , Fanelli F , Fava GA , Pasquali R . Psychological aspects of hyperandrogenic states in late adolescent and young women . Clin Endocrinol (Oxf) . 2015 ; 83 ( 6 ): 872 – 878 .
Styne DM , Arslanian SA , Connor EL , Farooqi IS , Murad MH , Silverstein JH , Yanovski JA . Pediatric obesity—assessment, treatment, and prevention: an Endocrine Society clinical practice guideline . J Clin Endocrinol Metab . 2017 ; 102 ( 3 ): 709 – 757 .
Legro RS , Arslanian SA , Ehrmann DA , Hoeger KM , Murad MH , Pasquali R , Welt CK ; Endocrine Society . Diagnosis and treatment of polycystic ovary syndrome: an Endocrine Society clinical practice guideline . J Clin Endocrinol Metab . 2013 ; 98 ( 12 ): 4565 – 4592 .
Goldenring J , Cohen E . Getting into adolescents HEADS . Contemp Pediatr . 1988 ; 5 ( 7 ): 75 – 90 .
Raja-Khan N , Agito K , Shah J , Stetter CM , Gustafson TS , Socolow H , Kunselman AR , Reibel DK , Legro RS . Mindfulness-based stress reduction in women with overweight or obesity: a randomized clinical trial . Obesity (Silver Spring) . 2017 ; 25 ( 8 ): 1349 – 1359 .
Dokras A , Witchel SF . Are young adult women with polycystic ovary syndrome slipping through the healthcare cracks ? J Clin Endocrinol Metab . 2014 ; 99 ( 5 ): 1583 – 1585 .
Abbott DH . Neuronal androgen receptor: molecular gateway to polycystic ovary syndrome ? Proc Natl Acad Sci USA . 2017 ; 114 ( 16 ): 4045 – 4047 .
Puttabyatappa M , Padmanabhan V . Ovarian and extra-ovarian mediators in the development of polycystic ovary syndrome . J Mol Endocrinol . 2018 ; 61 ( 4 ): R161 – R184 .
Email alerts
Citing articles via.
- About the Endocrine Society
- Advertising and Corporate Services
- Journals Career Network
Affiliations
- Online ISSN 2472-1972
- Copyright © 2024 Endocrine Society
- About Oxford Academic
- Publish journals with us
- University press partners
- What we publish
- New features
- Open access
- Institutional account management
- Rights and permissions
- Get help with access
- Accessibility
- Advertising
- Media enquiries
- Oxford University Press
- Oxford Languages
- University of Oxford
Oxford University Press is a department of the University of Oxford. It furthers the University's objective of excellence in research, scholarship, and education by publishing worldwide
- Copyright © 2024 Oxford University Press
- Cookie settings
- Cookie policy
- Privacy policy
- Legal notice
This Feature Is Available To Subscribers Only
Sign In or Create an Account
This PDF is available to Subscribers Only
For full access to this pdf, sign in to an existing account, or purchase an annual subscription.
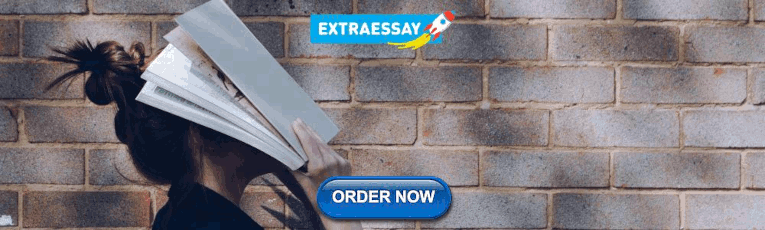
IMAGES
VIDEO
COMMENTS
1. Introduction. Polycystic ovary syndrome (PCOS) is a heterogeneous endocrine disorder that impacts many women of the reproductive age worldwide [].This syndrome is often associated with enlarged and dysfunctional ovaries, excess androgen levels, resistance to insulin, etc. [].It is estimated that approximately every 1 in 10 women face PCOS before menopause and struggle with its complications [].
Polycystic o vary syndrome (PCOS) is one of most common female endocrine d isorder t hat affects 6-15% of the female population. Women wit h. PCOS have a hormonal imbalance and metabolism pro ...
Download PDF. One in ten women of reproductive age is likely to have polycystic ovary syndrome (PCOS), a complex endocrine disorder that affects reproductive, metabolic, cardiovascular and ...
Introduction. Polycystic ovary syndrome (PCOS) is a complex reproductive, metabolic, and psychological condition that affects women's health and quality of life across the life course [1].Despite the high prevalence of PCOS of between 8% and 13%, depending on the population studied and definitions used [2, 3], care provision remains fragmented between health disciplines often resulting in ...
PDF | Polycystic ovary syndrome (PCOS), which affects 5-20% of women in their reproductive age, is the most common endocrinopa-thy affecting women... | Find, read and cite all the research you ...
1. Introduction. Polycystic ovarian syndrome (PCOS) is a heterogeneous endocrine disorder distinguished by the manifestation of ovarian cysts, anovulation, and endocrine variation that severely impact the life of a woman (Escobar-Morreale, 2018, Franks, 1995).The disturbance in the reproductive hormones like LH, FSH, estrogen, testosterone interrupts the normal menstrual cycle and would lead ...
Polycystic ovary syndrome (PCOS) is a complex endocrine and metabolic disorder, typically characterized by anovulation, infertility, obesity, insulin resistance, and polycystic ovaries. Lifestyle or diet, environmental pollutants, genetics, gut dysbiosis, neuroendocrine alterations, and obesity are among the risk factors that predispose females to PCOS. These factors might contribute to ...
Background Polycystic ovary syndrome (PCOS) is a common gynecological endocrine disease that has a great impact on women's physical and mental health. It is a burden to social and patients' economy. In recent years, researchers' understanding of PCOS has reached a new level. However, many PCOS reports have different directions, and overlapping phenomena exist. Therefore, clarifying the ...
Polycystic ovary syndrome (PCOS) is the major endocrine related disorder in young age women. Physical appearance, menstrual irregularity as well as infertility are considered as a sole cause of mental distress affecting health-related quality of life (HRQOL). This prospective case-control study was conducted among 100 PCOS and 200 healthy control cases attending tertiary care set up of AIIMS ...
Polycystic ovary syndrome (PCOS) is a heterogeneous endocrine disorder that impacts many women of the reproductive age worldwide [1]. This syndrome is often associated with enlarged and dysfunctional ovaries, excess androgen levels, resistance to insulin, etc. [2]. It is estimated that approximately every 1 in 10 women face PCOS before ...
Abstract. Polycystic ovary syndrome is a heterogeneous endocrine disorder that affects about one in 15 women worldwide. The major endocrine disruption is excessive androgen secretion or activity ...
Polycystic ovary syndrome (PCOS) is one of the most common female endocrine disorders. It is recognized by the presence of enlarged ovaries with multiple small cysts and a hypervascularized, androgen secreting stroma. This syndrome is characterized by menstrual abnormalities, infertility, obesity, excess hair growth, acanthosis nigricans and acne.
Polycystic ovary syndrome (PCOS) is a common endocrine disorder characterized by chronic ovulation dysfunction and overabundance of androgens; it affects 6-20% of women of reproductive age. PCOS involves various pathophysiological factors, and affected women usually have significant insulin resistance (IR), which is a major cause of PCOS. IR and compensatory hyperinsulinaemia have differing ...
Millets have been found to positively impact glycemic control, insulin sensitivity, and hormonal balance, which are crucial factors in PCOS pathophysiology, and offer a sustainable and accessible solution for PCOS management, promoting better dietary adherence and overall health outcomes. Polycystic Ovary Syndrome (PCOS) is a prevalent endocrine disorder affecting reproductive-aged women ...
Abstract. PCOD or PCOS is a condition that affects women's ovaries, the reproductive organs that produce progesterone and estrogen hormones that help in regulating the menstrual cycle and also ...
PCOS develops during the early pubertal years [].However, most relevant information has been accrued through clinical studies involving adult women in which referral bias focuses on investigation of the more severe phenotypes [].Preclinical models involving animal and in vitro studies supplement clinical investigation and benefit from other approaches to study this complex disorder.
View a PDF of the paper titled ReALM: Reference Resolution As Language Modeling, by Joel Ruben Antony Moniz and 7 other authors. View PDF HTML (experimental) Abstract: Reference resolution is an important problem, one that is essential to understand and successfully handle context of different kinds. This context includes both previous turns ...
Mixture-of-Depths:Dynamicallyallocatingcomputeintransformer-basedlanguagemodels thenumberoftokensinasequencethatcanparticipateinablock'scomputations(i.e.,self-