Henry Gustav Molaison: The Curious Case of Patient H.M.
Erin Heaning
Clinical Safety Strategist at Bristol Myers Squibb
Psychology Graduate, Princeton University
Erin Heaning, a holder of a BA (Hons) in Psychology from Princeton University, has experienced as a research assistant at the Princeton Baby Lab.
Learn about our Editorial Process
Saul Mcleod, PhD
Editor-in-Chief for Simply Psychology
BSc (Hons) Psychology, MRes, PhD, University of Manchester
Saul Mcleod, PhD., is a qualified psychology teacher with over 18 years of experience in further and higher education. He has been published in peer-reviewed journals, including the Journal of Clinical Psychology.
On This Page:
Henry Gustav Molaison, known as Patient H.M., is a landmark case study in psychology. After a surgery to alleviate severe epilepsy, which removed large portions of his hippocampus , he was left with anterograde amnesia , unable to form new explicit memories , thus offering crucial insights into the role of the hippocampus in memory formation.
- Henry Gustav Molaison (often referred to as H.M.) is a famous case of anterograde and retrograde amnesia in psychology.
- H. M. underwent brain surgery to remove his hippocampus and amygdala to control his seizures. As a result of his surgery, H.M.’s seizures decreased, but he could no longer form new memories or remember the prior 11 years of his life.
- He lost his ability to form many types of new memories (anterograde amnesia), such as new facts or faces, and the surgery also caused retrograde amnesia as he was able to recall childhood events but lost the ability to recall experiences a few years before his surgery.
- The case of H.M. and his life-long participation in studies gave researchers valuable insight into how memory functions and is organized in the brain. He is considered one of the most studied medical and psychological history cases.
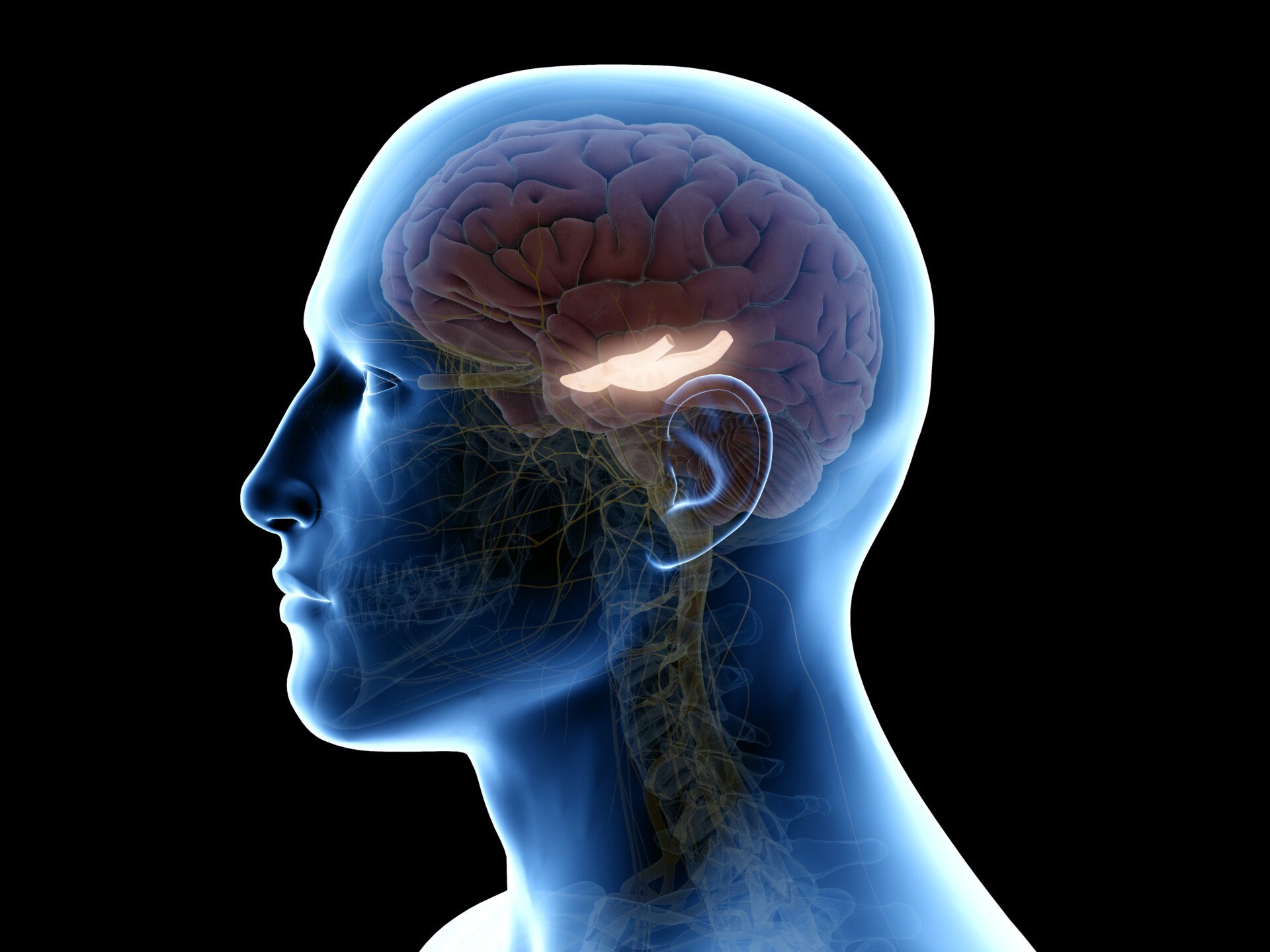
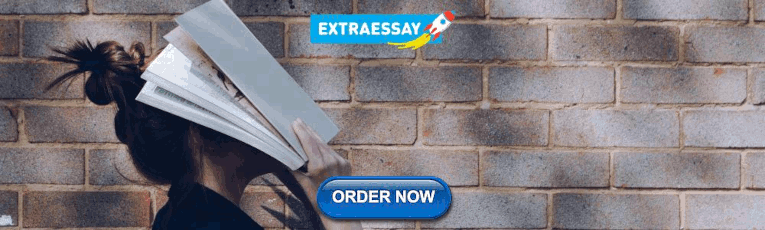
Who is H.M.?
Henry Gustav Molaison, or “H.M” as he is commonly referred to by psychology and neuroscience textbooks, lost his memory on an operating table in 1953.
For years before his neurosurgery, H.M. suffered from epileptic seizures believed to be caused by a bicycle accident that occurred in his childhood. The seizures started out as minor at age ten, but they developed in severity when H.M. was a teenager.
Continuing to worsen in severity throughout his young adulthood, H.M. was eventually too disabled to work. Throughout this period, treatments continued to turn out unsuccessful, and epilepsy proved a major handicap and strain on H.M.’s quality of life.
And so, at age 27, H.M. agreed to undergo a radical surgery that would involve removing a part of his brain called the hippocampus — the region believed to be the source of his epileptic seizures (Squire, 2009).
For epilepsy patients, brain resection surgery refers to removing small portions of brain tissue responsible for causing seizures. Although resection is still a surgical procedure used today to treat epilepsy, the use of lasers and detailed brain scans help ensure valuable brain regions are not impacted.
In 1953, H.M.’s neurosurgeon did not have these tools, nor was he or the rest of the scientific or medical community fully aware of the true function of the hippocampus and its specific role in memory. In one regard, the surgery was successful, as H.M. did, in fact, experience fewer seizures.
However, family and doctors soon noticed he also suffered from severe amnesia, which persisted well past when he should have recovered. In addition to struggling to remember the years leading up to his surgery, H.M. also had gaps in his memory of the 11 years prior.
Furthermore, he lacked the ability to form new memories — causing him to perpetually live an existence of moment-to-moment forgetfulness for decades to come.
In one famous quote, he famously and somberly described his state as “like waking from a dream…. every day is alone in itself” (Squire et al., 2009).
H.M. soon became a major case study of interest for psychologists and neuroscientists who studied his memory deficits and cognitive abilities to better understand the hippocampus and its function.
When H.M. died on December 2, 2008, at the age of 82, he left behind a lifelong legacy of scientific contribution.
Surgical Procedure
Neurosurgeon William Beecher Scoville performed H.M.’s surgery in Hartford, Connecticut, in August 1953 when H.M. was 27 years old.
During the procedure, Scoville removed parts of H.M.’s temporal lobe which refers to the portion of the brain that sits behind both ears and is associated with auditory and memory processing.
More specifically, the surgery involved what was called a “partial medial temporal lobe resection” (Scoville & Milner, 1957). In this resection, Scoville removed 8 cm of brain tissue from the hippocampus — a seahorse-shaped structure located deep in the temporal lobe .
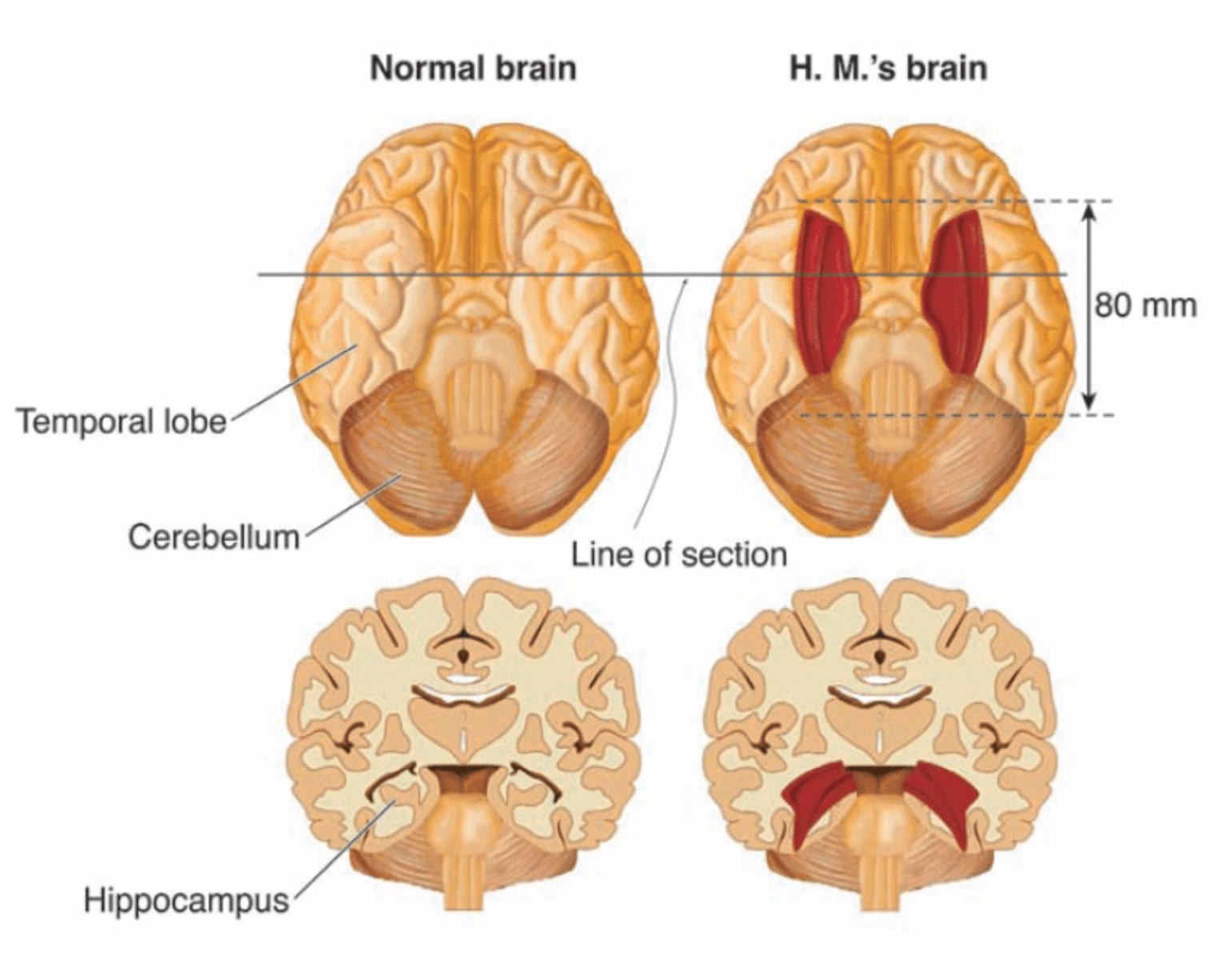
Bilateral resection of the anterior temporal lobe in patient HM.
Further research conducted after this removal showed Scoville also probably destroyed the brain structures known as the “uncus” (theorized to play a role in the sense of smell and forming new memories) and the “amygdala” (theorized to play a crucial role in controlling our emotional responses such as fear and sadness).
As previously mentioned, the removal surgery partially reduced H.M.’s seizures; however, he also lost the ability to form new memories.
At the time, Scoville’s experimental procedure had previously only been performed on patients with psychosis, so H.M. was the first epileptic patient and showed no sign of mental illness. In the original case study of H.M., which is discussed in further detail below, nine of Scoville’s patients from this experimental surgery were described.
However, because these patients had disorders such as schizophrenia, their symptoms were not removed after surgery. In this regard, H.M. was the only patient with “clean” amnesia along with no other apparent mental problems.
H.M’s Amnesia
H.M.’s apparent amnesia after waking from surgery presented in multiple forms. For starters, H.M. suffered from retrograde amnesia for the 11-year period prior to his surgery.
Retrograde describes amnesia, where you can’t recall memories that were formed before the event that caused the amnesia. Important to note, current research theorizes that H.M.’s retrograde amnesia was not actually caused by the loss of his hippocampus, but rather from a combination of antiepileptic drugs and frequent seizures prior to his surgery (Shrader 2012).
In contrast, H.M.’s inability to form new memories after his operation, known as anterograde amnesia, was the result of the loss of the hippocampus.
This meant that H.M. could not learn new words, facts, or faces after his surgery, and he would even forget who he was talking to the moment he walked away.
However, H.M. could perform tasks, and he could even perform those tasks easier after practice. This important finding represented a major scientific discovery when it comes to memory and the hippocampus. The memory that H.M. was missing in his life included the recall of facts, life events, and other experiences.
This type of long-term memory is referred to as “explicit” or “ declarative ” memories and they require conscious thinking.
In contrast, H.M.’s ability to improve in tasks after practice (even if he didn’t recall that practice) showed his “implicit” or “ procedural ” memory remained intact (Scoville & Milner, 1957). This type of long-term memory is unconscious, and examples include riding a bike, brushing your teeth, or typing on a keyboard.
Most importantly, after removing his hippocampus, H.M. lost his explicit memory but not his implicit memory — establishing that implicit memory must be controlled by some other area of the brain and not the hippocampus.
After the severity of the side effects of H.M.’s operation became clear, H.M. was referred to neurosurgeon Dr. Wilder Penfield and neuropsychologist Dr. Brenda Milner of Montreal Neurological Institute (MNI) for further testing.
As discussed, H.M. was not the only patient who underwent this experimental surgery, but he was the only non-psychotic patient with such a degree of memory impairment. As a result, he became a major study and interest for Milner and the rest of the scientific community.
Since Penfield and Milner had already been conducting memory experiments on other patients at the time, they quickly realized H.M.’s “dense amnesia, intact intelligence, and precise neurosurgical lesions made him a perfect experimental subject” (Shrader 2012).
Milner continued to conduct cognitive testing on H.M. for the next fifty years, primarily at the Massachusetts Institute of Technology (MIT). Her longitudinal case study of H.M.’s amnesia quickly became a sensation and is still one of the most widely-cited psychology studies.
In publishing her work, she protected Henry’s identity by first referring to him as the patient H.M. (Shrader 2012).
In the famous “star tracing task,” Milner tested if H.M.’s procedural memory was affected by the removal of the hippocampus during surgery.
In this task, H.M. had to trace an outline of a star, but he could only trace the star based on the mirrored reflection. H.M. then repeated this task once a day over a period of multiple days.
Over the course of these multiple days, Milner observed that H.M. performed the test faster and with fewer errors after continued practice. Although each time he performed the task, he had no memory of having participated in the task before, his performance improved immensely (Shrader 2012).
As this task showed, H.M. had lost his declarative/explicit memory, but his unconscious procedural/implicit memory remained intact. Given the damage to his hippocampus in surgery, researchers concluded from tasks such as these that the hippocampus must play a role in declarative but not procedural memory.
Therefore, procedural memory must be localized somewhere else in the brain and not in the hippocampus.
H.M’s Legacy
Milner’s and hundreds of other researchers’ work with H.M. established fundamental principles about how memory functions and is organized in the brain.
Without the contribution of H.M. in volunteering the study of his mind to science, our knowledge today regarding the separation of memory function in the brain would certainly not be as strong.
Until H.M.’s watershed surgery, it was not known that the hippocampus was essential for making memories and that if we lost this valuable part of our brain, we would be forced to live only in the moment-to-moment constraints of our short-term memory .
Once this was realized, the findings regarding H.M. were widely publicized so that this operation to remove the hippocampus would never be done again (Shrader 2012).
H.M.’s case study represents a historical time period for neuroscience in which most brain research and findings were the result of brain dissections, lesioning certain sections, and seeing how different experimental procedures impacted different patients.
Therefore, it is paramount we recognize the contribution of patients like H.M., who underwent these dangerous operations in the mid-twentieth century and then went on to allow researchers to study them for the rest of their lives.
Even after his death, H.M. donated his brain to science. Researchers then took his unique brain, froze it, and then in a 53-hour procedure, sliced it into 2,401 slices which were then individually photographed and digitized as a three-dimensional map.
Through this map, H.M.’s brain could be preserved for posterity (Wb et al., 2014). As neuroscience researcher Suzanne Corkin once said it best, “H.M. was a pleasant, engaging, docile man with a keen sense of humor, who knew he had a poor memory but accepted his fate.
There was a man behind the data. Henry often told me that he hoped that research into his condition would help others live better lives. He would have been proud to know how much his tragedy has benefitted science and medicine” (Corkin, 2014).
Corkin, S. (2014). Permanent present tense: The man with no memory and what he taught the world. Penguin Books.
Hardt, O., Einarsson, E. Ö., & Nader, K. (2010). A bridge over troubled water: Reconsolidation as a link between cognitive and neuroscientific memory research traditions. Annual Review of Psychology, 61, 141–167.
Scoville, W. B., & Milner, B. (1957). Loss of recent memory after bilateral hippocampal lesions . Journal of neurology, neurosurgery, and psychiatry, 20 (1), 11.
Shrader, J. (2012, January). HM, the man with no memory | Psychology Today. Retrieved from, https://www.psychologytoday.com/us/blog/trouble-in-mind/201201/hm-the-man-no-memory
Squire, L. R. (2009). The legacy of patient H. M. for neuroscience . Neuron, 61 , 6–9.

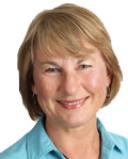
HM, the Man with No Memory
Henry molaison (hm) taught us about memory by losing his..
Posted January 16, 2012 | Reviewed by Jessica Schrader
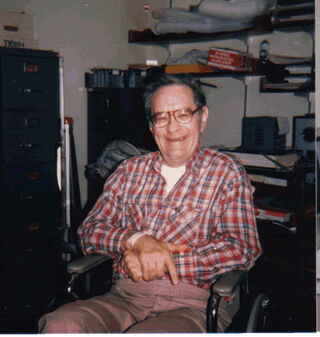
Henry Molaison, known by thousands of psychology students as "HM," lost his memory on an operating table in a hospital in Hartford in August 1953. He was 27 years old and had suffered from epileptic seizures for many years.
William Beecher Scoville, a Hartford neurosurgeon , stood above an awake Henry and skilfully suctioned out the seahorse-shaped brain structure called the hippocampus that lay within each temporal lobe. Henry would have been drowsy and probably didn't notice his memory vanishing as the operation proceeded.
The operation was successful in that it significantly reduced Henry's seizures, but it left him with a dense memory loss. When Scoville realized his patient had become amnesic, he referred him to the eminent neurosurgeon Dr. Wilder Penfield and neuropsychologist Dr. Brenda Milner of Montreal Neurological Institute (MNI), who assessed him in detail. Up until then, it had not been known that the hippocampus was essential for making memories, and that if we lose both of them we will suffer a global amnesia. Once this was realized, the findings were widely publicized so that this operation to remove both hippocampi would never be done again.
Penfield and Milner had already been conducting memory experiments on other patients and they quickly realized that Henry's dense amnesia, his intact intelligence , and the precise neurosurgical lesions made him the perfect experimental subject. For 55 years, Henry participated in numerous experiments, primarily at Massachusetts Institute of Technology (MIT), where Professor Suzanne Corkin and her team of neuropsychologists assessed him.
Access to Henry was carefully restricted to less than 100 researchers (I was honored to be one of them), but the MNI and MIT studies on HM taught us much of what we know about memory. Of course, many other patients with memory impairments have since been studied, including a small number with amnesias almost as dense as Henry's, but it is to him we owe the greatest debt. His name (or initials!) has been mentioned in almost 12,000 journal articles, making him the most studied case in medical or psychological history. Henry died on December 2, 2008, at the age of 82. Until then, he was known to the world only as "HM," but on his death his name was revealed. A man with no memory is vulnerable, and his initials had been used while he lived in order to protect his identity .
Henry's memory loss was far from simple. Not only could he make no new conscious memories after his operation, he also suffered a retrograde memory loss (a loss of memories prior to brain damage) for an 11-year period before his surgery. It is not clear why this is so, although it is thought this is not because of his loss of the hippocampi on both sides of his brain. More likely it is a combination of his being on large doses of antiepileptic drugs and his frequent seizures prior to his surgery. His global amnesia for new material was the result of the loss of both hippocampi, and meant that he could not learn new words, songs or faces after his surgery, forgot who he was talking to as soon as he turned away, didn't know how old he was or if his parents were alive or dead, and never again clearly remembered an event, such as his birthday party, or who the current president of the United States was.
In contrast, he did retain the ability to learn some new motor skills, such as becoming faster at drawing a path through a picture of a maze, or learning to use a walking frame when he sprained his ankle, but this learning was at a subconscious level. He had no conscious memory that he had ever seen or done the maze test before, or used the walking frame previously.
We measure time by our memories, and thus for Henry, it was as if time stopped when he was 16 years old, 11 years before his surgery. Because his intelligence in other non-memory areas remained normal, he was an excellent experimental participant. He was also a very happy and friendly person and always a delight to be with and to assess. He never seemed to get tired of doing what most people would think of as tedious memory tests, because they were always new to him! When he was at MIT, between test sessions he would often sit doing crossword puzzles, and he could do the same ones again and again if the words were erased, as to him it was new each time.
Henry gave science the ultimate gift: his memory. Thousands of people who have suffered brain damage, whether through accident, disease or a genetic quirk, have given similar gifts to science by agreeing to participate in psychological, neuropsychological, psychiatric and medical studies and experiments, and in some cases by gifting their brains to science after their deaths. Our knowledge of brain disease and how the normal mind works would be greatly diminished if it were not for the generosity of these people and their families (who are frequently also involved in interviews, as well as transporting the "patient" back and forth to the psychology laboratory). After Henry's death, his brain was dissected into 2,000 slices and digitized as a three-dimensional brain map that could be searched by zooming in from the whole brain to individual neurons. Thus, his tragically unique brain has been preserved for posterity.
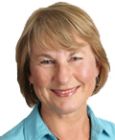
Jenni Ogden, Ph.D. , clinical neuropsychologist and author of Trouble in Mind, taught at the University of Auckland.
- Find a Therapist
- Find a Treatment Center
- Find a Psychiatrist
- Find a Support Group
- Find Teletherapy
- United States
- Brooklyn, NY
- Chicago, IL
- Houston, TX
- Los Angeles, CA
- New York, NY
- Portland, OR
- San Diego, CA
- San Francisco, CA
- Seattle, WA
- Washington, DC
- Asperger's
- Bipolar Disorder
- Chronic Pain
- Eating Disorders
- Passive Aggression
- Personality
- Goal Setting
- Positive Psychology
- Stopping Smoking
- Low Sexual Desire
- Relationships
- Child Development
- Therapy Center NEW
- Diagnosis Dictionary
- Types of Therapy
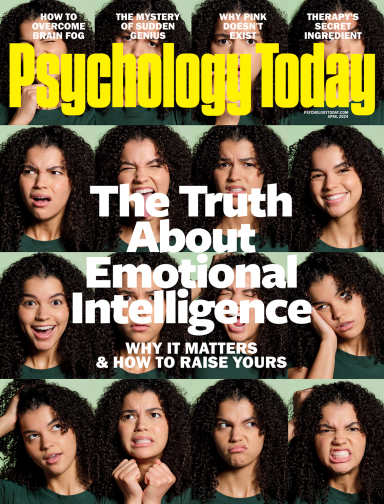
Understanding what emotional intelligence looks like and the steps needed to improve it could light a path to a more emotionally adept world.
- Coronavirus Disease 2019
- Affective Forecasting
- Neuroscience
- Search Menu
- Advance articles
- Editor's Choice
- Continuing Education
- Author Guidelines
- Submission Site
- Open Access
- Why publish with this journal?
- About Archives of Clinical Neuropsychology
- About the National Academy of Neuropsychology
- Journals Career Network
- Editorial Board
- Advertising and Corporate Services
- Self-Archiving Policy
- Dispatch Dates
- Journals on Oxford Academic
- Books on Oxford Academic
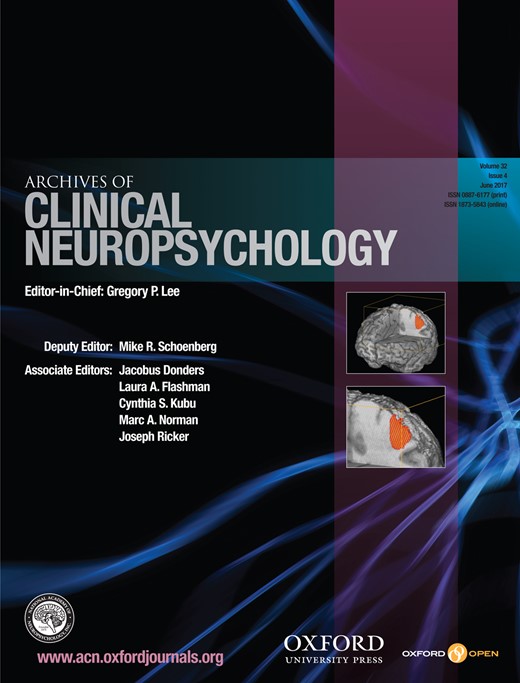
Article Contents
Influential case studies, psychosurgery and asylums, temporal lobectomy, controversy, author notes.
- < Previous
Remembering H.M.: Review of “PATIENT H.M.: A Story of Memory, Madness, and Family Secrets”
- Article contents
- Figures & tables
- Supplementary Data
David W. Loring, Bruce Hermann, Remembering H.M.: Review of “PATIENT H.M.: A Story of Memory, Madness, and Family Secrets”, Archives of Clinical Neuropsychology , Volume 32, Issue 4, June 2017, Pages 501–505, https://doi.org/10.1093/arclin/acx041
- Permissions Icon Permissions
Although many influential case reports in neuropsychology exist ( Code, Wallesch, Joanette, & Lecours, 1996 ), there are certain patients who stand out because, based upon the historical zeitgeist in which their brain injuries occurred and the attention that those cases received, their neurobehavioral deficits and circumstances of their injury greatly altered our knowledge of brain-behavior relationships.
Among the most famous of these cases is Phineas Gage, the railroad foreman whose personality dramatically changed following frontal lobe injury in 1848 from an accidental explosion that thrust his tamping iron through his skull. Gage's survival after such a serious injury was a surprise, but Gage's contribution to clinical neuroscience was his significant personality change, aptly described by his physicians with the pithy observation, “Gage was no longer Gage” ( Macmillan, 2000 ). Although his personality changes were well documented soon after the accident, much of Gage's long-term outcome may have been exaggerated for entertainment value ( Macmillan & Lena, 2010 ). Thus, the lasting neurobehavioral effects of Gage's frontal lobe injury and how the deficits may have evolved over time remain clouded in the historical record due to the absence independent scientific characterization.
The second patient is Louis Victor Leborgne, whose expressive language disturbance from a left frontal lobe lesion was described in 1861 by the famous French neurologist Pierre Paul Broca. Monsieur Tan, as he was informally called because “tan tan” was his typical verbal output, retained his capacity to understand commands. The deficits of Monsieur Tan, supported by subsequent cases, demonstrated that language could be fractionated into different components associated with distinct brain regions, and that language was predominately a function of the left brain. Monsieur Tan's contribution, however, was in no small part due to Broca's distinguished reputation as a physician and scientist since localized language effects had been previously described by Jean-Baptiste Bouillard ( Sondhaus & Finger, 1988 ).
The third and most studied of these three cases is patient Henry Molaison (H.M.). H.M. suffered a dense and persistent anterograde amnesia following bilateral medial temporal lobectomy in 1953 to treat intractable epilepsy ( Scoville & Milner, 1957 ). His scientific fame derives from the dramatic demonstration of the critical role that the mesial temporal lobe structures play in learning and memory. Unlike Gage and Monsieur Tan, H.M.’s brain injury was iatrogenic, being an unanticipated adverse event associated with the surgical treatment of his epilepsy. Another important difference is that H.M.’s surgery injury occurred in what can broadly be considered to be the beginning of the modern era of neuroscience ( Shepherd, 2010 ). Thus, his cognitive abilities were subjected to formal characterization with extensive neuropsychological testing over five decades, providing a much richer characterization of his clinical semiology compared to Gage or Monsieur Tan.
H.M.’s amnesia framed how the neuroscience community would eventually conceptualize basic memory mechanisms, beginning with Brenda Milner's early demonstration that multiple memory systems exist such that declarative and procedural memory are readily dissociable ( Milner, 1965 ). Clinically, H.M.’s amnesia meaningfully influenced pre-operative epilepsy surgery protocols across the world. After several additional cases of post-surgical amnesia developed following unilateral temporal lobectomy, it was hypothesized that the functional reserve of the contralateral temporal lobe was insufficient to support the encoding of new memories following resection of the epileptogenic temporal lobe and mesial structures, and multiple methods for characterizing functional hippocampus status were developed ( Milner, 1975 ). What remains poorly reported in standard textbooks, however, is the historical context in which the decision to undergo epilepsy surgery was made, the blurring between experimental clinical techniques and informed consent, and the profound effects on H.M.’s quality of life.
To provide this broad historical context of H.M., Luke Dittrich has published PATIENT H.M.: A Story of Memory, Madness, and Family Secrets ( Dittrich, 2016a ). This is far from a narrative review of H.M.’s contributions to understanding memory, and it is also not a typical biography. However, as the grandson of William Beecher Scoville, MD, the neurosurgeon who performed H.M.’s operation and a prolific practitioner of psychosurgery, Dittrich provides a unique “insider” perspective and captivating description of that era's medical zeitgeist that could not be easily achieved without such a personal relationship. In fact, much of the book does not directly involve H.M.’s life story, but rather, the management of significant psychiatric disease prior to the development of neuroleptics.
Scoville's neurosurgical practice primarily involved surgery for psychiatric indications rather than epilepsy surgery. The early development of psychosurgery's goals is exemplified with a quote from the 19th century physician Dr. Gottleib Burckhardt, who resected undifferentiated brain areas, that illustrates the depersonalization of patients with psychiatric disease: “Mrs. B. has changed from a dangerous and excited demented person to a quiet demented one” (p. 79). It was in late 1935, after listening to the report of operations on two chimpanzees, that Egas Moniz oversaw the first in his series of approximately 20 frontal leucotomies/lobotomies. This series significantly influenced Walter Freeman (neurologist) and James Watts (neurosurgeon) who initially worked together performing prefrontal lobotomies. The distinct approaches to frontal lobotomy developed by Scoville and Freeman also provide a striking contrast in how to best decrease the institutional burden of psychiatric disease. Although Scoville is described as an adventurer who liked expensive sports cars, he was a meticulous neurosurgeon with painstaking preparation before and during all surgical cases. Freeman's enthusiastic efforts to expand the use of frontal lobotomy was reflected by his technique in which an ice pick, inserted through the orbital sockets to a depth of approximately 3 inches, was moved back and forth for frontal disconnection before repeating the procedure on the opposite side. As practiced by Freeman, frontal lobotomy required approximately 15 min to complete, could be performed without a surgeon or an operating room, and multiple procedures could be easily performed in a single day. “Any reasonably competent psychiatrist (could be trained) to perform the ice-pick lobotomy in an afternoon” (p. 151). One can go elsewhere for the complete story of Freeman, his activities and their aftermath, which has been covered by others including the exquisite text by Elliot Valenstein (1986) .
Dittrich's concerns regarding psychiatric therapies during this era are not limited to psychosurgery. His grandmother, Scoville's wife, experienced a breakdown sometime after their marriage, suffered a brittle psychiatric course, and was institutionalized at the Hartford Institute of Living while her husband was director of neurosurgery there and was performing lobotomies at both the Institute of Living and Hartford Hospital. A variety of harsh non-surgical but unproven psychiatric treatments were used that included: (1) Continuous hydrotherapy in which patients were submerged in a tub with only their heads protruding through a small aperture. (2) Pyrotherapy in which patients were placed in a small copper coffin appearing device that, over a repeated treatment period of days, would elevate core temperatures to 105–106 °C. (3) Electric Shock Therapy. In response to patients’ fears about these therapies, treatment names were changed. “Since these treatments produce states of unconsciousness akin to normal slumber … we are adopting the names that are more truly descriptive of these treatments—INSULIN, METRAZOL, and ELECTRIC SLEEP” (p. 73). Karl Pribram, who was head of research at the Institute of Living at that time, claimed that Scoville had performed a frontal leucotomy on his wife, although Dittrich could not independently substantiate that assertion.
A recurring theme throughout PATIENT HM is the concept embodied by the Hippocratic Oath of “ primum non nocer ” (first, do no harm) as it contrasts with “ melius anceps remedium quam nullum” (it is better to do something than nothing). The tension between these approaches lies at the foundation of modern informed consent in which risks and benefits are carefully weighed as part of the decision-making processes prior to treatment initiation or when deciding to participate in clinical research. Informed consent discussion is not restricted to psychosurgery, shock therapies, or H.M. The rationale for informed consent includes the development of surgical treatment for vesicovaginal fistula by J. Marion Sims during the mid-19th century that was conducted on his slaves prior to application to white women, to the U.S. Public Health Service Tuskegee Syphilis Experiment in the 1930s, and the history of the Doctors Trial at Nuremberg after World War II resulting in the Nuremberg Code.
Scoville was a practitioner of psychosurgery rather than epilepsy surgery, and prior to H.M.’s surgery, Scoville had performed multiple bilateral temporal lobectomies for psychiatric indications. Although he describes H.M.’s surgery as an “experimental operation,” he also states that the procedure was considered due to H.M.’s seizure frequency and severity despite adequate medical therapy, and that surgery was “carried out with the understanding and approval of the patient and his family” ( Scoville & Milner, 1957 ).
By the time of H.M.’s surgery in 1953, the first reported series of temporal lobectomies for epilepsy had been published from the Montreal Neurological Institute (MNI) ( Penfield & Flanigin, 1950 ). Dittrich describes the important contributions of Wilder Penfield in epilepsy surgery development that ranged from identification of motor and sensory homunculi to how Penfield established a multidisciplinary and state of the art institute by including neurology, electrophysiology, and neuropsychology colleagues. It was in this context that Penfield hired Brenda Milner. A brief biography of Milner's early life is presented in which she designed psychological aptitude tests at Cambridge University during World War II before moving to Montreal and enrolling at McGill University as a graduate student of Donald Hebb.
Although H.M.’s surgery was not performed at the MNI, Milner's neuropsychological testing of epilepsy surgery patients at the MNI made her arguably the most appropriate individual to characterize H.M.’s memory impairment. The first formal scientific presentation of H.M.’s amnesia was published in 1957 by Scoville and Milner although his “very grave, recent memory loss” was described in 1953 at a meeting of the Harvey Cushing Society ( Scoville, 1954 ). However, the 1957 report also contains formal testing on additional temporal lobectomies performed on “seriously ill schizophrenic patients who had failed to respond to other forms of treatment” (p. 11), two of whom also developed significant amnesia following bitemporal resection. Orbital undercutting was extended to include the medial temporal lobes in the “hope that still greater psychiatric benefit might be obtained” (p. 11). The significant psychiatric disease of these patients decreased clinical awareness of memory change without Milner's formal testing given that “the psychotic patients were for the most part too disturbed before operation for finer testing of higher mental functions to be carried out” (p. 12). Thus, the extent of the memory impairment was unknown due to the significant overlaying psychiatric disease in the non-epilepsy patients on whom Dr. Scoville had performed bitemporal resection prior to H.M.
Scoville was sufficiently enthusiastic about the procedure to travel to teach other surgeons the technique. Interesting is mention of Scoville's trip to Manteno State Hospital, an extremely large psychiatric facility located south of Chicago in Manteno, Illinois. Here faculty from the University of Illinois were performing anterior temporal lobectomies that included hippocampal resection, something not undertaken by Percival Bailey in his series in Chicago. Dittrich mentions another severely amnestic case (D.C.) as an outcome of Scoville's surgery at Mantero, a physician from Chicago with a premorbid IQ of 122. He was evaluated postoperatively with the resulting amnesia, comparable to H.M., confirmed by Brenda Milner. This case was apparently very unsettling to Scoville.
It is impossible to review PATIENT HM without consideration of outside events that occurred after its publication. The New York Times Magazine published a book excerpt on August 3, 2016, beginning with interviews with H.M. illustrating the magnitude and severity of his memory impairment, briefly discussing post-mortem brain ownership disagreements between the University of California at San Diego and Massachusetts Institute of Technology, presenting background material on the tension between research groups surrounding manuscript preparation describing an previously unknown lesion in H.M.’s frontal lobe that was detected at autopsy, and discussing how H.M.’s court-appointed guardian was identified. The excerpt concludes with interview quotations from Dr. Suzanne Corkin, who was the principal investigator of H.M.’s amnesia since 1977 following the death of Hans-Lukas Teuber. Again, in an interesting personal twist, Corkin lived across the street from the Scovilles, and was one of Dittrich's mother's best friends during their childhood and adolescence.
After The Times’ excerpt appeared, MIT and other organizations quickly issued statements disputing Dittrich's assertions and conclusions ( Eichenbaum & Kensinger, 2016 ; MIT News Office, 2016 ). The main points of contention included: (1) allegation that research records were or would be destroyed or shredded, (2) allegation that the finding of an additional lesion in left orbitofrontal cortex was suppressed, and (3) allegation that there was something inappropriate in the selection of (the conservator) as Mr. Molaison's guardian. In addition, a letter signed by over 200 scientists supporting Corkin dated August 5, 2017 was sent to The Times ( DiCarlo et al., 2016 ) asserting that Dittrich's claims were untrue.
Part of the interest in the quick response by the scientific community presumably was that Corkin died on May 24, 2016 prior to the book's publication and was unable to respond to these concerns. While Dittrich (2016b) has directly addressed each of the MIT concerns, their response has nevertheless led many of our colleagues and students to assume that Dittrich's book was incendiary, and whose entire story should not be believed.
While the interested reader will examine both sides of the argument (see Vyse, 2016 ), there is no evidence to suggest that any of Dittrich's factual allegations are wrong. Thus, there are two important points to consider when deciding if this controversy should make otherwise interested individuals pass on reading the book. First, in response to the assertion that research records were shredded, some have suggested Corkin's use of “shredding” was either colloquial or referred to material no longer considered relevant. Corkin is explicit in her description of data shredding in the audio clip of her interview that Dittrich posted ( Dittrich, 2016b ). Certainly, the presence of many files in a storage room says nothing about whether any files had been shredded, particularly since there has never apparently been a comprehensive catalog of the material established. Non-published information can still inform our understanding of H.M.’s clinical course as demonstrated by Dittrich's observation that H.M. had a significant memory impairment prior to surgery, a fact that had not been formally published. Similarly, non-significant findings or “failed experiments” also demonstrate a broader representation of functions either affected or unchanged following surgery. As Dittrich notes, Corkin was a “meticulous investigator, keeping careful notes” (p. 270), and these notes have both scientific and historical value.
H.M.’s legal guardianship merits greater discussion compared to disagreements about scientific ownership and publication disputes, however, which unfortunately are sufficiently common that university committees exist to address such conflicts. Conservatorship, however, is central to this story because it affects the informed consent for H.M.’s research participation, as well as influencing the final disposition of H.M.’s brain after autopsy. Similar to research study reporting standards, the nature of informed consent has evolved over the course of H.M.’s research participation. Consequently, the absence of any conservator or formal consent process early in H.M.’s research participation reflected generally accepted standards at that time. In 1992, an independent conservator was sought for H.M. to mitigate against unintended conflict of interest by H.M.’s investigators, reflecting greater overall awareness of the importance of informed consent.
The eventual conservator was a son of a former landlady of H.M. Dittrich provides evidence that, in contrast to formal court filings, the conservator was not a relative, and that one of H.M.’s relatives was a first cousin sharing H.M.’s last name (Frank Molaison). We will never fully know how the various points are intertwined or even if H.M.’s relatives had been contacted and were not interested in assuming the role of conservator, and part of this controversy is that Corkin's perspective on Dittrich's claims cannot be obtained. Nevertheless, Dittrich's reporting these issues are neither irrelevant nor inappropriate. Careful consideration of H.M.‘s ability to provide informed consent, and how conservatorship is established in circumstances in which research subjects cannot fully consent, will increase awareness of ambiguities that will allow future researchers to confidently ensure full and appropriate consent is obtained prior to research participation.
Most of the book presents a non-controversial narrative, however, and that was not adequately captured by The Times’ excerpt. What we found to be particularly enjoyable in this book is that it provides new details on the contours of H.M.’s life. Prior to H.M.’s death, there were few personal details known to the scientific community, so it should not be surprising that much of this book's appeal is due to its biographical content reporting a variety of details about H.M.’s past. Upon hearing of H.M.’s death, the initial knowledge of his full name was both exciting but then also associated with some sense of guilt and dismay as if suddenly becoming privy to secret information that had been inadvertently revealed. We enjoyed reading about H.M.’s confusion of The Beatles with The Rolling Stones when examining a photograph, but then accurately spelling B-E-A-T-L-E-S rather than beetles, but there are many others throughout the book such as H.M.’s thick New England accent. When asked “Who, or what, is Sue Corkin,” H.M. replied “She was a … well, a senator.” The book also describes frequent angry outbursts including physical harm to himself, which contrasts with the typical H.M. description of his being agreeable and passive, and it is interesting to speculate whether this behavior might have been related to the orbitofrontal damage identified during autopsy. These pieces of personal information help humanize H.M. rather than simply being either a research subject or clinical syndrome. A particularly poignant comment by H.M. was his statement that “every day is alone in itself. Whatever enjoyment I've had, and whatever sorrow I've had” (p. 375).
Despite the controversies that arose after publication of The Times’ excerpt, or perhaps because of them, this book provides a unique glimpse into the blurring of experimental therapy and research during the mid-20th century, motivations for finding treatments for psychiatrically intractable patients prior to the development of neuroleptics, as well as professional interactions and conflicts that may arise in collaborative research settings. Unlike Gage and Monsieur Tan, the depth of clinical research and the modern era in which he lived not only makes H.M. one of the most influential case studies in clinical neuroscience, but also provides one of the most compelling individual stories about how unanticipated surgical effects robbed H.M. of the capacity to form meaningful and lasting relationships with others due to the inability to form new memories. Though clearly not a textbook, and undeniably chatty at times, this is a volume that neuropsychologists at all levels of training and experience, and particularly those with interests in the history of medicine, will enjoy reading and remembering for a long time.
PATIENT H.M: A Story of Memory, Madness, and Family Secrets received the 2017 The PEN/E. O. Wilson Literary Science Writing Award. We thank Kimford J. Meador for his helpful comments on an earlier draft of this review.
Code , C. , Wallesch , C. W. , Joanette , Y. , & Lecours , A. R. ( 1996 ). Classic cases in neuropsychology . New York : Psychology Press .
Google Scholar
Google Preview
DiCarlo , J. J. , Kanwisher , N. , Gabrieli , J. D. E. , Adcock , R. A. , Addis , D. R. , Aggleton , J. P. , et al. . ( 2016 ). Letter to the Editor of the New York Times Magazine. Retrieved from https://bcs.mit.edu/news-events/news/letter-editor-new-york-times-magazine .
Dittrich , L. ( 2016 b). Questions & Answers about “Patient H.M.” Retrieved frrom https://medium.com/@lukedittrich/questions-answers-about-patient-h-m-ae4ddd33ed9c#.apelhqx85.
Dittrich , L. ( 2016 a). PATIENT H.M.: A story of memory, madness, and family secrets . New York : Random House .
Eichenbaum , H. , & Kensinger , E. ( 2016 ). In defense of Suzanne Corkin. Retrieved from https://www.psychologicalscience.org/observer/in-defense-of-suzanne-corkin#.WKIOVE3rvL8 .
Macmillan , M. ( 2000 ). Restoring Phineas Gage: A 150th retrospective . Journal of the History of Neurosciences , 9 (1), 46 – 66 .
Macmillan , M. , & Lena , M. L. ( 2010 ). Rehabilitating Phineas Gage . Neuropsychological Rehabilitation , 20 (5), 641 – 658 .
Milner , B. ( 1965 ). Visually guided maze learning in man: Effects of bilateral hippocampal, bilateral frontal, and unilateral cerebral lesions . Neuropsychologia , 3 , 317 – 338 .
Milner , B. ( 1975 ). Psychological aspects of focal epilepsy and its neurosurgical management . Advances in Neurology , 8 , 299 – 321 .
MIT News Office ( 2016 ). Faculty at MIT and beyond respond forcefully to an article critical of Suzanne Corkin. Retrieved from http://news.mit.edu/2016/faculty-defend-suzanne-corkin-0809 .
Penfield , W. , & Flanigin , H. ( 1950 ). Surgical therapy of temporal lobe seizures . AMA Archives of Neurology & Psychiatry , 64 (4), 491 – 500 .
Scoville , W. B. ( 1954 ). The limbic lobe in man . Journal of Neurosurgery , 11 , 64 – 66 .
Scoville , W. B. , & Milner , B. ( 1957 ). Loss of recent memory after bilateral hippocampal lesions . Journal of Neurology, Neurosurgery & Psychiatry , 20 , 11 – 21 .
Shepherd , G. M. ( 2010 ). Creating modern neuroscience: The revolutionary 1950s . New York : Oxford University Press .
Sondhaus , E. , & Finger , S. ( 1988 ). Aphasia and the CNS from Imhotep to Broca . Neuropsychology , 2 (2), 87 – 119 .
Valenstein , E. ( 1986 ). Great and desperate cures: The rise and decline of psychosurgery and other radical treatments for mental Illness . New York : Basic Books .
Vyse , S. ( 2016 ). Consensus: Could two hundred scientists be wrong? Retrieved from http://www.csicop.org/specialarticles/show/consensus_could_two_hundred_scientists_be_wrong .
Email alerts
Citing articles via.
- Recommend to your Library
Affiliations
- Online ISSN 1873-5843
- Copyright © 2024 Oxford University Press
- About Oxford Academic
- Publish journals with us
- University press partners
- What we publish
- New features
- Open access
- Institutional account management
- Rights and permissions
- Get help with access
- Accessibility
- Advertising
- Media enquiries
- Oxford University Press
- Oxford Languages
- University of Oxford
Oxford University Press is a department of the University of Oxford. It furthers the University's objective of excellence in research, scholarship, and education by publishing worldwide
- Copyright © 2024 Oxford University Press
- Cookie settings
- Cookie policy
- Privacy policy
- Legal notice
This Feature Is Available To Subscribers Only
Sign In or Create an Account
This PDF is available to Subscribers Only
For full access to this pdf, sign in to an existing account, or purchase an annual subscription.
- Brain Development
- Childhood & Adolescence
- Diet & Lifestyle
- Emotions, Stress & Anxiety
- Learning & Memory
- Thinking & Awareness
- Alzheimer's & Dementia
- Childhood Disorders
- Immune System Disorders
- Mental Health
- Neurodegenerative Disorders
- Infectious Disease
- Neurological Disorders A-Z
- Body Systems
- Cells & Circuits
- Genes & Molecules
- The Arts & the Brain
- Law, Economics & Ethics
- Neuroscience in the News
- Supporting Research
- Tech & the Brain
- Animals in Research
- BRAIN Initiative
- Meet the Researcher
- Neuro-technologies
- Tools & Techniques
Core Concepts
- For Educators
- Ask an Expert
- The Brain Facts Book

Patient Zero: What We Learned from H.M.
- Published 16 May 2013
- Author Dwayne Godwin
- Source BrainFacts/SfN
Memory is our most prized human treasure. It defines our sense of self, and our ability to navigate the world. It defines our relationships with others – for good or ill – and is so important to survival that our gilled ancestors bear the secret of memory etched in their DNA. If you asked someone over 50 to name the things they most fear about getting older, losing one’s memory would be near the top of that list. There is so much worry over Alzheimer’s disease, the memory thief, that it is easy to forget that our modern understanding of memory is still quite young, less than one, very special lifespan.
Meet the Patient Zero of memory disorders, H.M.
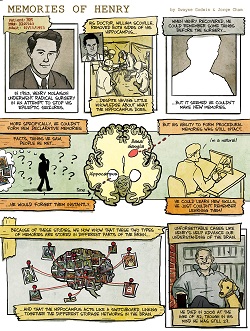
H.M. was the pseudonym of Henry Molaison, a man who was destined to change the way we think about the brain. Permanent Present Tense: The Unforgettable Life of the Amnesic Patient H.M. is a touching, comprehensive view of his life through the eyes of a researcher who also, in a sense, became part of his family.
The prologue opens with a conversation between the author, Suzanne Corkin, and Molaison in 1992. It reads a bit like a first meeting of two strangers, but then Corkin reveals a jarring truth: this meeting was one of many similar encounters they’d had over 30 years.
By now, if you’re interested in learning and memory you probably know the basics of Henry Molaison’s story. He had epilepsy from an early age that was thought to be acquired through head trauma from a bike accident (though apparently family members also had epilepsy). His surgeon, William B. Scoville (who in a remarkable twist, was a childhood neighbor of Suzanne Corkin) removed Henry’s hippocampus and amygdala in both hemispheres of his brain, in an attempt to control his seizures.
The results of the surgery are legendary. While Henry’s seizures were controlled, he suffered a type of profound anterograde amnesia that prevented him from encoding new memories, but spared certain details of his life leading up to the surgery. Henry would have no memory of those he worked with from day to day, or of new information he might encounter. The book’s title, “Permanent Present Tense”, describes his zen-like existence within the thirty or so seconds around the present moment, which was the limit of Henry’s short term memory.
If this book were a movie or video game, it would be said to be full of “Easter eggs”. There are vignettes and bits of unexpected information that add rich historical context to the state of knowledge in Molaison’s time. These include a digression on the history of neurosurgery, including the gruesome history of lobotomies and the advances brought to the field of neurosurgery by Wilder Penfield. In many ways, H.M.’s legend is a product of a unique scientific lineage – Scoville owed much to Penfield, who in turn trained under Charles Sherrington (he who gave “synapse” to the neuroscience lexicon), and Brenda Milner, who trained under Donald Hebb (who spawned our current notion of activity-dependent plasticity, embodied by the phrase, “cells that fire together wire together”).
The book also reminds us that H.M. was not the first amnesic patient produced through neurosurgical interventions to treat intractable epilepsy, but he was by far the most studied. The book conveys a sense of wonder at the accomplishments of scientists and physicians, charting terra incognita with scalpels, electrical probes and psychological test batteries.
Corkin recounts Henry Molaison’s early life, including key events - like a childhood plane ride that Henry remembered after his surgery - with gentle but thorough prose. Some of these details come from personal conversations with Henry, while others are the result of careful reporting and research.
The book is an accessible master class in learning and memory, with details and key milestones culled from Corkin’s decades of experience as a memory researcher. The details are not so burdensome as to be esoteric, nor so simple as to be trivial. The book gives only a brief overview of the growing field of knowledge about the cellular mechanisms supporting learning and memory (which might be lost on a casual reader), but this is wisely offset by the details of functional anatomy gleaned from Henry and other patients, and a solid explanation of how we encode, store and retrieve memories.
A light, scholarly tone is maintained throughout the book, but it occasionally brushes up against the deeply personal. It’s difficult to hear Henry’s story and not wonder (or actually, worry) about how it was to live as Henry lived, trapped in the moment. Corkin is reassuring on this point:
“When we consider how much of the anxiety and pain of daily life stems from attending to our long-term memories and worrying about and planning for the future, we can appreciate why Henry lived much of his life with little stress…in the simplicity of a world bounded by thirty seconds.”[p. 75].
In other words, the very thing that might cause Henry to fret about his condition was missing. Henry’s tragedy, it seems, is in the mind of the beholder. Another interesting passage concerns Henry’s moods – which were usually happy and content, but could occasionally be sad or uneasy. This is interesting given the removal during his surgery of a major part of his emotional processing circuitry of the brain, called the amygdala.
Henry Molaison’s anterograde amnesia was practically absolute. However, something not often noted is that he would occasionally surprise those studying him by recalling something he should not be able to remember - for example, colored pictures, or details of celebrities he had heard about after his surgery. Corkin reasons that a bit of spared medial temporal lobe may explain these moments.
Henry was amnesic, but he was not without memory. Through careful behavioral testing, various types of memory function could be uncovered, including recognition memory for having seen images that could persist for months. Corkin suggests that this “memory for the familiar” may have been of some comfort as he navigated what would have otherwise been a confusing experience of reality. New technologies like computers, for example, could be incorporated into his view of the world and did not appear to be jarring to him as would be expected if his capacity for recognizing the familiar did not exist.
Another key discovery from Henry was the finding that he had retained the ability to form non-declarative memories, which took the form of improvement in motor skills. This separate memory system depended on regions of the basal ganglia and motor cortex, which were spared in Henry’s surgery. Testing could improve his performance in the motor task, but his impaired declarative memory system didn’t allow him to remember taking the tests – he could be surprised by his own improvement. Along with his simple recognition memory, motor memory helped smooth challenges Henry faced as he aged, such as learning to use a walker.
Other forms of memory in which Henry showed improvement were in picture completion, where he was able to identify a picture from fragments over a series of sessions, and priming, where previously presented words could prime recognition on presenting fragments of the words. And while Henry is best known for anterograde amnesia, and is sometimes portrayed as having intact memories of things and events before the surgery, he also possessed a partial retrograde amnesia, especially for autobiographical events that happened two years before the surgery - he had only fragmented memories from before that two year window.
Did Henry Molaison have a sense of self? While his was not a fully integrated personality, he possessed “beliefs, desires and values” and seemed capable of a full set of emotions – even without his amygdalae. His view of his own appearance did not seem to cause him distress, even though his estimate of his own age could vary widely. His impairment prevented him from formulating future plans. His basic decency shines through the narrative.
Henry died in 2008 at the age of 82. His brain was scanned postmortem, and extracted for further anatomical analysis . Coming full circle from one of his remaining childhood memories of his first ride, Corkin describes her last wistful goodbye to Henry’s brain as it was conveyed by his final plane ride back to the west coast, where his brain was sliced up into thin sections for new studies. Perhaps the most documented and studied research subject in neuroscience continues to provide vast amounts of data to further our knowledge.
Henry once remarked about his testing, of which he never seemed to become bored since he carried little from one session to the next: “It’s a funny thing – you just live and learn.” He then went on to provide a poignant turn in the familiar phrase: “I’m living, and you’re learning.”
Though he’s no longer living, we’re still learning from Henry.
Permanent Present Tense is a rare look at an amazing mind, whose study formed the basis of our modern science of memory.
Corkin, Suzanne. Permanent Present Tense: The Unforgettable Life of the Amnesic Patient, H.M. (Basic Books) May 14, 2013 | ISBN-10: 0465031595 | ISBN-13: 978-0465031597
Update 6/7/2013: NPR interview with Suzanne Corkin on H.M .
Update 1/30/2014: Report on anatomical and histological findings from Henry Molaison: Postmortem examination of patient H.M.’s brain based on histological sectioning and digital 3D reconstruction . J Annese, NM Schenker-Ahmed, H Bartsch, P Maechler, C Sheh, N Thomas, J Kayano, A Ghatan, N Bresler, MP Frosch, R Klaming & S Corkin. Nature Communications 5, Article number: 3122
Update 7/6/2016: Statement on informed consent transmitted to me by Suzanne Corkin
About the Author
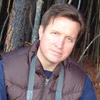
Dwayne Godwin
Dwayne Godwin is a Professor of Neurobiology and Neurology at the Wake Forest University School of Medicine, where he studies epilepsy, sensory processing, withdrawal and PTSD. He coauthors a comic strip on brain topics for Scientific American Mind .
CONTENT PROVIDED BY
BrainFacts/SfN
Also In Learning & Memory
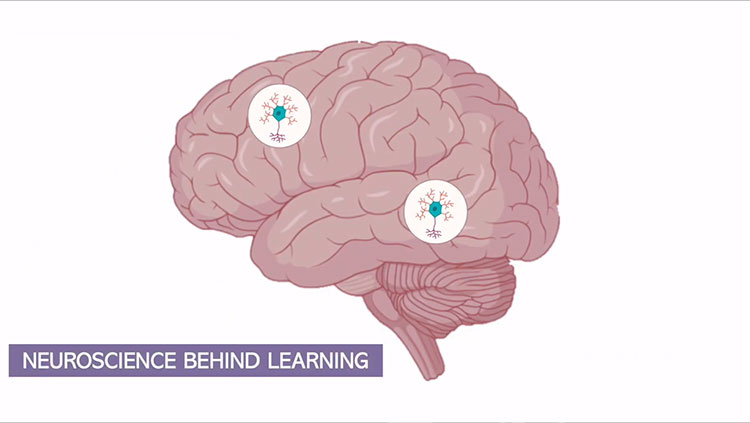
Popular articles on BrainFacts.org
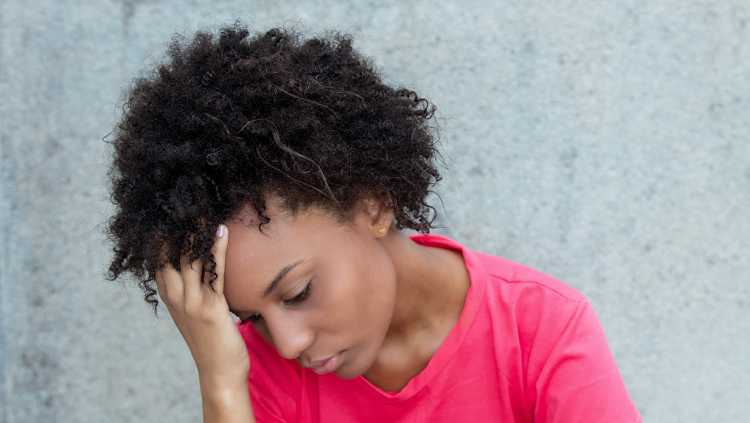
Ask An Expert
Ask a neuroscientist your questions about the brain.
Submit a Question
BrainFacts Book
Download a copy of the newest edition of the book, Brain Facts: A Primer on the Brain and Nervous System.
A beginner's guide to the brain and nervous system.
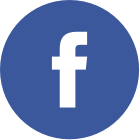
SUPPORTING PARTNERS
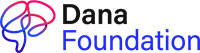
- Privacy Policy
- Accessibility Policy
- Terms and Conditions
- Manage Cookies
Some pages on this website provide links that require Adobe Reader to view.
Thank you for visiting nature.com. You are using a browser version with limited support for CSS. To obtain the best experience, we recommend you use a more up to date browser (or turn off compatibility mode in Internet Explorer). In the meantime, to ensure continued support, we are displaying the site without styles and JavaScript.
- View all journals
- My Account Login
- Explore content
- About the journal
- Publish with us
- Sign up for alerts
- Open access
- Published: 28 January 2014
Postmortem examination of patient H.M.’s brain based on histological sectioning and digital 3D reconstruction
- Jacopo Annese 1 , 2 ,
- Natalie M. Schenker-Ahmed 1 , 2 ,
- Hauke Bartsch 1 , 2 ,
- Paul Maechler 1 , 2 ,
- Colleen Sheh 1 , 2 ,
- Natasha Thomas 1 na1 ,
- Junya Kayano 1 na1 ,
- Alexander Ghatan 1 na1 ,
- Noah Bresler 1 ,
- Matthew P. Frosch 3 ,
- Ruth Klaming 1 , 2 &
- Suzanne Corkin 4
Nature Communications volume 5 , Article number: 3122 ( 2014 ) Cite this article
83k Accesses
102 Citations
483 Altmetric
Metrics details
- Brain imaging
Modern scientific knowledge of how memory functions are organized in the human brain originated from the case of Henry G. Molaison (H.M.), an epileptic patient whose amnesia ensued unexpectedly following a bilateral surgical ablation of medial temporal lobe structures, including the hippocampus. The neuroanatomical extent of the 1953 operation could not be assessed definitively during H.M.’s life. Here we describe the results of a procedure designed to reconstruct a microscopic anatomical model of the whole brain and conduct detailed 3D measurements in the medial temporal lobe region. This approach, combined with cellular-level imaging of stained histological slices, demonstrates a significant amount of residual hippocampal tissue with distinctive cytoarchitecture. Our study also reveals diffuse pathology in the deep white matter and a small, circumscribed lesion in the left orbitofrontal cortex. The findings constitute new evidence that may help elucidate the consequences of H.M.’s operation in the context of the brain’s overall pathology.
Similar content being viewed by others
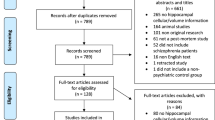
Hippocampal volume and hippocampal neuron density, number and size in schizophrenia: a systematic review and meta-analysis of postmortem studies
Maxwell J. Roeske, Christine Konradi, … Alan S. Lewis
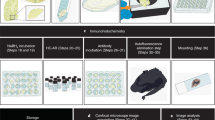
Unraveling human adult hippocampal neurogenesis
Miguel Flor-García, Julia Terreros-Roncal, … María Llorens-Martín
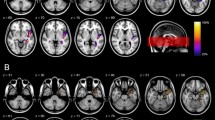
Multi-scale image analysis and prediction of visual field defects after selective amygdalohippocampectomy
Bastian David, Jasmine Eberle, … Theodor Rüber
Introduction
Henry G. Molaison, known in the medical literature as Patient H.M., began experiencing minor epileptic seizures when he was 10 years old and major seizures when he was 15. The underlying aetiology of his seizure disorder remains uncertain; a minor head injury earlier in his childhood may have been a contributing factor. The worsening seizures not only compromised his health but also disrupted his school performance and social life; as a young adult, epilepsy affected his ability to work and function independently. In 1953, because even very high doses of available anticonvulsant medications were ineffective, the 27-year-old underwent a neurosurgical procedure performed by William Beecher Scoville, with the intention of decreasing the impact of his seizures on his quality of life.
Following the surgery, in which portions of the medial temporal lobes (MTL) were resected bilaterally, H.M.’s seizure frequency was reduced, although he remained on anticonvulsant medication. There was, however, an unexpected and profound effect on his behaviour: after recovering from the operation, he was unable to recollect routine events during his hospital stay and did not recognize the staff that attended him assiduously. In spite of this obvious impairment, H.M.’s intellectual abilities and personality appeared to be unaffected 1 . His language and perceptual skills were intact; moreover, based on tests done 10 months after the operation, when the seizures had subsided, his IQ was above average 2 . His attentional and working memory capacities were normal, and he was able to hold items in mind by actively rehearsing them. He could not, however, consolidate and store this information in long-term memory. For example, he was able to carry on a conversation proficiently but several minutes later would be unable to remember having had the exchange or the person with whom he spoke. Further testing gradually revealed some forms of preserved learning and memory. Notably, he demonstrated intact motor skill learning 3 , 4 , classical conditioning 5 , perceptual learning 6 , and visuoperceptual priming 7 , although he was oblivious to the fact that he had repeatedly performed these tasks with the experimenter and had no declarative knowledge of the learning experience 8 , 9 , 10 . H.M. demonstrated this new procedural learning through performance but could not verbalize what he had learned. Researchers concluded that this nondeclarative learning relied on memory circuits separate from those in the MTL region and that it did not require conscious memory processes 10 .
H.M.’s semantic memory for the years preceding the operation was preserved, but he could not retrieve any episodic, autobiographical memories from that time. Instead, he conceptualized his memories of friends and experiences, relating only general knowledge about people and places 6 , 11 , 12 , 13 . Postoperatively, H.M. was profoundly impaired in learning new episodic and semantic information, but gradually over time he acquired a few new facts and snippets of knowledge about celebrities, likely due to repeated exposure to this information 14 , 15 . The defining deficit in H.M.’s case was an inability to form new declarative memories, a syndrome that became indelibly linked to the bilateral resection of the hippocampus and neighbouring MTL structures 16 .
H.M. made his debut into the medical literature as a case described in a seminal publication, which is one of the most cited articles in the medical literature 1 . His case acquired broad significance in the field largely because the neurological substrate of memory was unknown at the time of his operation and H.M. provided the first conclusive evidence for the involvement of the hippocampal complex. Scoville’s intraoperative impressions, however, were the only estimate of the extent of H.M.’s lesions.
Before H.M.’s surgery, Scoville published several illustrations of his surgical technique 16 , 17 ; these sketches showed how metal retractors were inserted through two trephine holes ∼ 1 inch above the orbits to lift the frontal lobes. Postoperative drawings reflected Scoville’s intent to carry out a symmetrical medial resection reaching as far as 8 cm from the midpoints of the tips of the temporal lobes 1 , 18 . This procedure would have removed the uncus, amygdala and the hippocampal complex, including the parahippocampal gyrus (based on the drawings, the ablation affected the anterior calcarine cortex, which contains the representation for the peripheral visual field) 1 . At the time of the operation it was not possible to evaluate postsurgical outcomes with neuroimaging; thus, Scoville’s drawings were only speculative.
The first modern CT scans of H.M.’s brain published in 1984 did not clearly reveal the nature and extent of tissue damage in the temporal lobes 2 ; however, a better view of H.M.’s brain was obtained in 1992 and 1993 using magnetic resonance imaging (MRI) 19 . MRI scans revealed that the lesion was symmetrical as Scoville had planned, but was less extensive than his estimate 19 . It included the medial temporal polar cortex, most of the amygdaloid complex and entorhinal cortex (EC), and about half of the rostro-caudal extent of the intraventricular segment of the hippocampal formation (dentate gyrus, hippocampus and subiculum). Portions of the ventral perirhinal cortex were spared, and the parahippocampal cortex appeared largely intact. The frontal, parietal and occipital cortices had a generally normal appearance, and neocortical atrophy was slight and consistent with H.M.’s age. The authors pointed out that any residual hippocampal tissue had been significantly deafferented by the removal of the EC and was, therefore, unlikely to have preserved function.
A higher-resolution series of MRI scans conducted when H.M. was in his mid-seventies revealed a number of age-related morphological changes: cortical thinning, atrophy of deep grey matter structures and a large volume of abnormal white matter (WM) and deep grey matter 20 . Most of these alterations appeared to be of recent origin, and were attributed to acquired medical conditions, including hypertension. The MRI scans collected in vivo lacked sufficient resolution to reveal the exact anatomical boundaries of the MTL lesions, and to characterize secondary changes.
H.M. died of respiratory failure on 2 December 2008. Direct examination of the brain, combined with postmortem imaging 21 , 22 , represented the opportunity to address the limitations of previous non-invasive MRI studies 19 , 20 and to provide a clear anatomical verification of the lesion and the pathologic state of surrounding areas.
Our goal was to create a detailed three-dimensional (3D) model of the whole brain from high-resolution anatomical images so that we could revisit, by virtual dissection, Scoville’s surgical procedure and localize the anatomical borders of the lesion in the temporal lobe. The data also supported accurate measurements of H.M.’s remaining hippocampus and assessment of the tissue at the microscopic level. Because it was critical to preserve an organized archive of images and histological slices to conduct retrospective studies, we designed the protocols and instrumentation necessary to collect and image a complete series of histological sections through the whole brain. Detailed mapping at the microscopic level and 3D measurements within the digital model of the brain showed that H.M. had retained a significant portion of the hippocampus that appears histologically intact in both hemispheres.
Dissection and tomographic imaging of the brain specimen
The ventral aspect of the brain, after the removal of the leptomeninges, clearly showed the scars of the operation on both hemispheres ( Fig. 1 ). On the surface, the right and left lesions appeared roughly symmetrical in length and in respect to anatomical landmarks; however, the full extent of the injury could only be determined upon dissection. Serial sectioning and direct tomographic imaging was performed in the coronal plane in alignment with the anterior and posterior commissures (AC-PC); these interhemispheric fibre bundles run perpendicular to the major axis of the brain and form the reference points for a widely-used standard radiologic stereotaxic system 23 . AC-PC alignment ensured that histological sections could be compared with previous scans of H.M.’s brain 19 , 20 , those of other patients 24 , 25 , 26 , 27 , and neuroimaging data from population-based studies 28 . Rigorous stereotaxic orientation also guaranteed that any interhemispheric differences in the length of the lesion or in the morphology of the spared hippocampus noted in the histological images reflected actual anatomical asymmetry rather than unconventional planes of section.
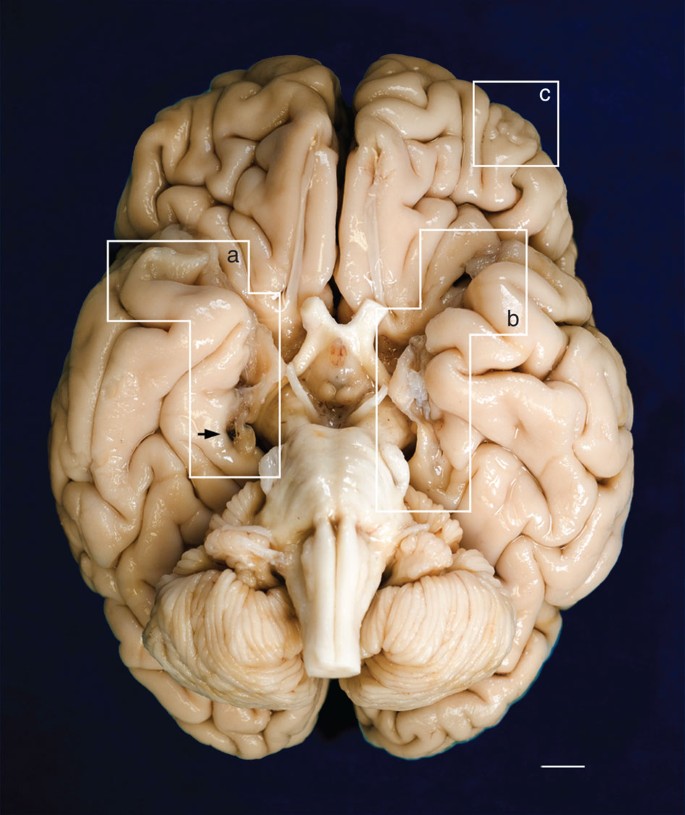
The fixed specimen was photographed after removal of the leptomeninges. Evidence of the surgical lesions in the temporal lobes is highlighted by white geometric contours (a, b). A mark produced by the oxidation of one of the surgical clips inserted by Scoville is visible on the parahippocampal gyrus of the right hemisphere (black arrow). (c) encloses a lesion in the orbitofrontal gyrus that affects the cortex and WM. Marked cerebellar atrophy is consistent with H.M.’s long-term treatment with phenytoin. Scale bar, 1 cm.
The results of our examination are based on 2,401 digital anatomical images and selected corresponding histological sections that were collected at an interval of 70 μm over the course of an uninterrupted 53-hour procedure. The series of digital images of the block’s surface was obtained using a digital camera mounted directly above the microtome stage. Volumetric reconstruction from these images was the basis for subsequent visualization and 3D measurements along arbitrary planes. The dissection of the brain was video-recorded and streamed live on the web to permit scientific scrutiny and to foster public engagement in the study 29 .
3D anatomical measurements in the MTL
Using the 3D measurement tools in AMIRA (FEI Visualization Science Group, Burlington, MA, USA) we calculated the distance in each hemisphere from the anterior tip of the temporal lobe to the posterior boundary of the surgical lesion on each side. This limit was marked by the most anterior coronal anatomical images that did not show any sign of disruption in the normal anatomy, which was confirmed in corresponding stained histological slices. The lesion followed a straight, but slightly oblique path relative to the long axis of each hemisphere; measured along this axis, its length was 54.5 mm and 44.0 mm in the left and right hemispheres, respectively. The value for the left hemisphere was consistent with earlier measurements made on H.M.’s MRI scans acquired in 1992–1993 (ref. 19 ) and 2002–2004 (ref. 20 ). The lesion in the right hemisphere as measured in our postmortem data was 7 mm shorter than in the above-mentioned reports that were based on in vivo imaging.
The borders of the surgical resection were clearly demarcated in the anatomical and histological images; the latter clearly showed that the WM underlying the excised medial temporal cortex was also damaged ( Fig. 2 ). We identified a small portion of the superior-most region of the EC in both hemispheres based on its distinctive cytoarchitecture; specifically, 0.03 cm 3 and 0.11 cm 3 for the left and right hemispheres, respectively (these values correspond approximately to 1.7% and 6.5% of the normal volume, based on published MRI estimates 30 ). Portions of the centromedial nucleus of the amygdala were also preserved ( Fig. 2h,i ).
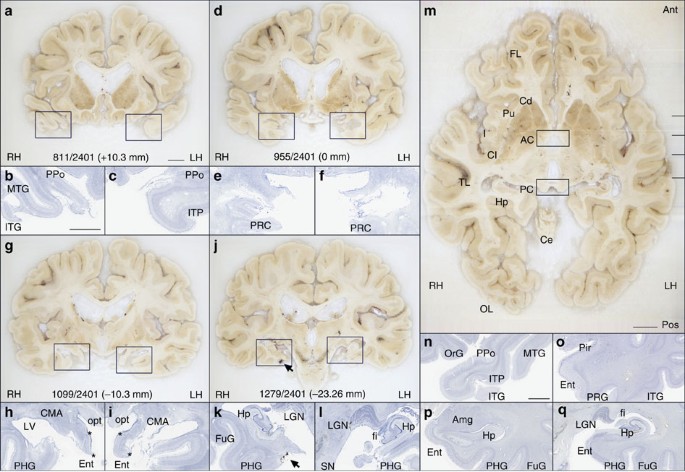
( a , d , g , j ) Cross-sectional anatomy of patient H.M.’s MTL shown at four different levels. The values below the tissue indicate the section number and in parenthesis, the distance from the origin of the standard coordinate system 23 (positive if the level is anterior to the anterior commissure, negative if posterior). Scale bar, 1 cm. ( b , c ), ( e , f ), ( h , i ), ( k , l ): close-up images acquired from thionin-stained tissue slices; these panels illustrate histological detail for the selection boxes in a , d , g , j , respectively. Scale bar, 5 mm. ( m ) Horizontal cross-sectional view reconstructed orthogonally from the original coronal images showing the correct alignment of the anterior and posterior commissures. ( n – q ): normal anatomy and histology of the MTL at the levels shown for the brain of patient H.M. The images were derived from brain slices belonging to a neurologically normal, age-matched individual. Scale bar, 5 mm. PPo, planum polare; MTG, middle temporal gyrus; ITG, inferior temporal gyrus; ITP, inferior temporopolar cortex; PRC, perirhinal cortex; CMA, centromedial amygdala; opt, optic tract; LV, lateral ventricle; PHG, parahippocampal gyrus; Ent, entorhinal cortex; LGN, lateral geniculate nucleus; Hp, hippocampus; FuG, fusiform gyrus; fi, fimbria; SN, substantia nigra; FL, frontal lobe; Cd, caudate; Pu, putamen; I, insula; AC, anterior commissure; Cl, claustrum; TL, temporal lobe; PC, posterior commissure; Ce, cerebellum; OL, occipital lobe; OrG, orbital gyrus; Pir, pirifom cortex; Amg, amygdala. The asterisks delimit the entorhinal cortex.
The extent of the spared hippocampus measured along the horizontal axis of the brain (which in our study coincided with the AC–PC line) was 23.6 mm in the left hemisphere and 24.3 mm in the right. Our measurements included the alveus and the thin band of WM that encapsulates the posterior end of the hippocampus; the fimbria was excluded from our delineations. The discrepancy between these new values and those obtained from earlier MRI data (19 and 22 mm, respectively) is small and can be explained by differences in the quality of the image data 19 . These linear measurements, however, do not fully account for the geometry of H.M.’s spared hippocampus. The high-resolution anatomical volume that we created from microtome images allowed us to inspect the MTL from multiple angles, revealing that the posterior hippocampus was bent steeply in the dorso-medial direction ( Fig. 3 ). This curvature is a normal feature of the periventricular portion of the hippocampus in the human brain, while the most anterior portion, which is immediately posterior to the amygdala and adjacent to the EC, is aligned to the horizontal plane (or major axis) of the hemisphere.
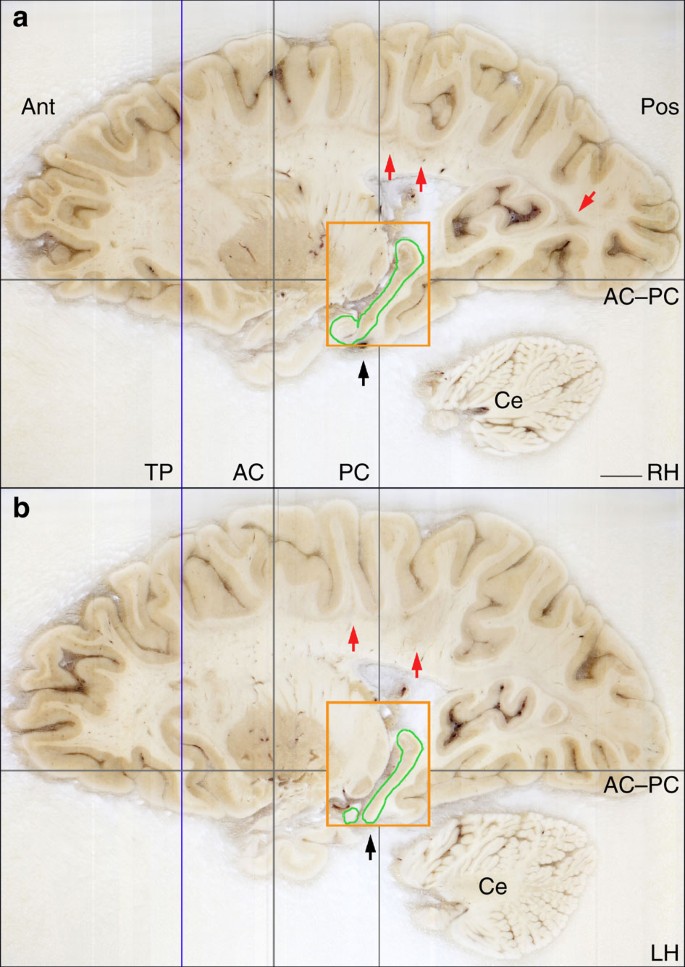
The grey lines intersect at the origin of the origins of the standard coordinate system 23 used for the orientation of the specimen. The blue line indicates the most anterior level of the temporal lobes (the temporal poles, which are damaged are not shown in this image). The orange rectangle represents the bounding box that contains the posterior spared hippocampus (outlined in green), the widest extent of which is at a more medial level than this image shows. The black arrows identify the level at which a surgical clip was positioned on a blood vessel. The red arrows indicate the presence of lesions in the subcortical WM. AC, plane of the anterior commissure; PC, plane of the posterior commissure; AC–PC, ideal plane at the level of both the AC and PC; Ce, cerebellum; LG, lateral geniculate nucleus; Pu, putamen; TP, temporal pole; RH, right hemisphere; LH, left hemisphere. Scale bar, 1 cm.
Measuring the dorso-ventral oblique length of the posterior segment of H.M.’s hippocampus rather than along the AC–PC line produced higher values, specifically, 36.0 mm for the left hemisphere and 40.0 mm for the right. The values were greater when our calculations took curvature into account: in this case, the actual ‘geodesic’ length of the preserved hippocampus amounted to 45.4 mm in the left hemisphere and 47.2 mm in the right ( Fig. 4 ).
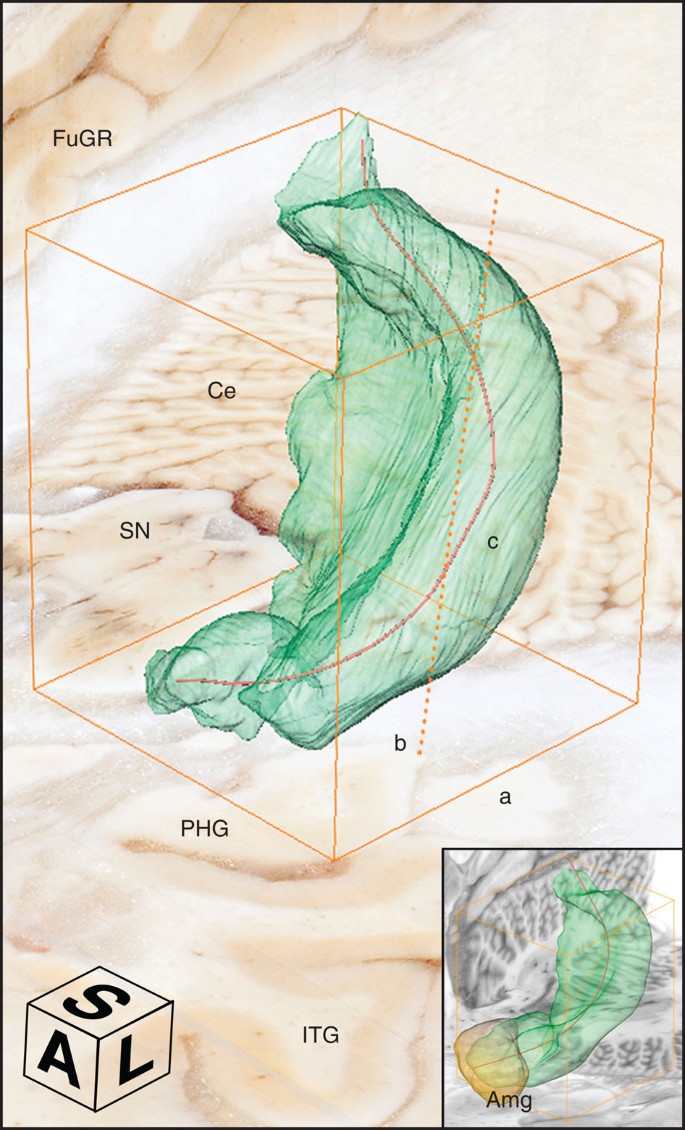
The orange bounding box delimits the dimensions of the structure; different segments indicate different measurements; a: anterior-to-posterior extent in the coronal plane, b (dotted): major diagonal axis, c: anatomical length. The compass cube (A: anterior, L: lateral, S: superior) also functions as scale bar: 5 mm. The insert at the bottom right shows a similarly constructed model from the brain of a neurologically normal subject (78-year-old female donor; volume of the right hippocampus=3.04 mm 3 ; total brain weight 1,278). Ce, cerebellum; FuGR, fusiform gyrus of right hemisphere; IT, inferior temporal gyrus; SN, substantia nigra; Amg, amygdala.
The fact that multiple results can be obtained based on different measuring criteria makes it necessary to establish a clear terminology to describe the anatomy of H.M.’s lesion and the remaining hippocampus in relation to previous reports. In the context of this communication, we refer to the extent of H.M.’s hippocampus and related structures as their linear span in the rostro-caudal direction. This measure can be obtained simply by multiplying the number of tomographic images by the interval between them (that is, slice thickness). The anatomical length of the hippocampus is different and depends on its 3D shape and orientation in the brain. With this distinction in mind, our findings can be more easily reconciled with previous reports that were based on low-resolution scans, where only the extent of the hippocampus was actually measured 19 .
The level of sampling and image quality afforded by the current study represents a significant advance over the first clinical MRI that was performed with H.M. 19 . At that time, the two-dimensional (2D) MRI sequences produced 4–5-mm thick slabs with a 1-mm gap between each slice and an in-plane resolution slightly better than 1 mm per voxel; a T1-weighted 3D MRI scan was also acquired and produced non-isotropic voxels (1 × 1 × 3.2 mm). In these early scans, the boundaries of the posterior hippocampus were blurred by partial volume effects (the presence of multiple tissue types or structures in single large MRI voxels).
In a more recent MRI study with H.M., Salat et al. 20 calculated the volume of tissue ascribed to the posterior hippocampus (voxel size: 1 mm × 1 mm × 1.3 mm) and obtained values of 0.65 cm 3 for the left hemisphere and 0.88 cm 3 for the right. We repeated these measurements based on manual delineations made on three orthogonal views of the digital anatomical reconstruction, and determined that 2.02 cm 3 of hippocampal tissue (including the cornu ammonis, the dentate gyrus and the subiculum) was spared in the left hemisphere and 1.96 cm 3 in the right. The comparison should be interpreted with caution in view of the fact that sufficient normative data on hippocampal volume based on our new postmortem methodology is not yet available. The labelled fields representing the posterior hippocampus in each hemisphere were used to compute triangulated surface models for visualization and shape analyses ( Fig. 4 ).
Regularly spaced tissue sections through the brain were selected at an interval of 1.26 mm; these were mounted on large-format glass slides (5 × 7 in) and stained using thionin. Nissl staining with thionin showed preservation of neuronal cell bodies in CA1, CA2, CA3, CA4 and the subiculum of the hippocampal formation, posterior to the surgical lesion ( Fig. 5 ).
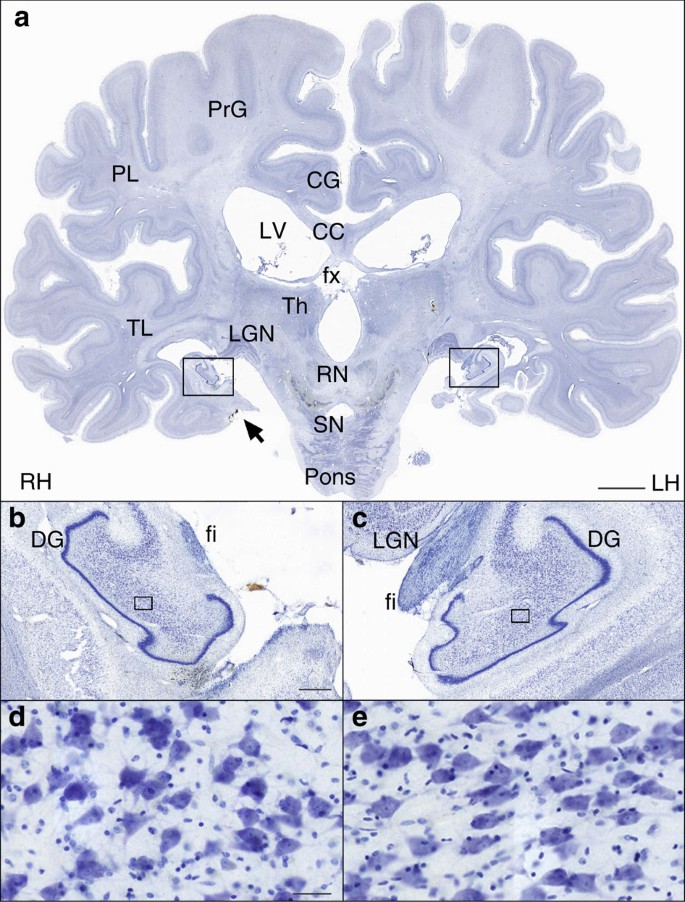
( a ) Whole section. Scale bar, 1 cm. ( b , c ) Higher-magnification cross-sectional image of the dentate gyrus of the spared hippocampus. The fimbria is visible below the lateral geniculate nucleus. Scale bar, 1 mm. ( d , e ) × 20 magnification image of neurons in the CA4 region of the hippocampus (in the location of the box). Scale bar, 50 μm. PrG, precentral gyrus; PL, parietal lobe; CG, cingulate gyrus; LV, lateral ventricle; cc, corpus callosum; fx, fornix; Th, thalamus; TL, temporal lobe; LG, lateral geniculate nucleus; RN, red nucleus; SN, substantia nigra; DG, dentate gyrus; fi, fimbria.
Given that H.M. required surgery because of epilepsy and that there was partial response to the surgical intervention, it is of interest that the typical neuropathologic hallmarks associated with idiopathic temporal lobe epilepsy (granule cell dispersion in the dentate gyrus, neuronal loss from the pyramidal cell layer particularly in area CA4) were not present in the residual hippocampus.
Pathologic anatomy beyond the MTL
While the general size and cortical folding of the cerebral hemispheres appeared normal for an individual of H.M.’s age, multiple WM lesions consistent with lacunar infarctions were present. Cerebellar atrophy was also evident, likely a consequence of long-term exposure to Dilantin (phenytoin sodium), which was part of H.M.’s seizure management pre- and postoperatively 31 , 32 .
Review of the MRI scans and histological sections demonstrated a spectrum of additional lesions in the WM ( Fig. 6 ). We also discovered a small focal lesion in the left lateral orbital gyrus, which was visible on the surface ( Fig. 1 c) and involved both cortex and underlying WM ( Fig. 7 ).
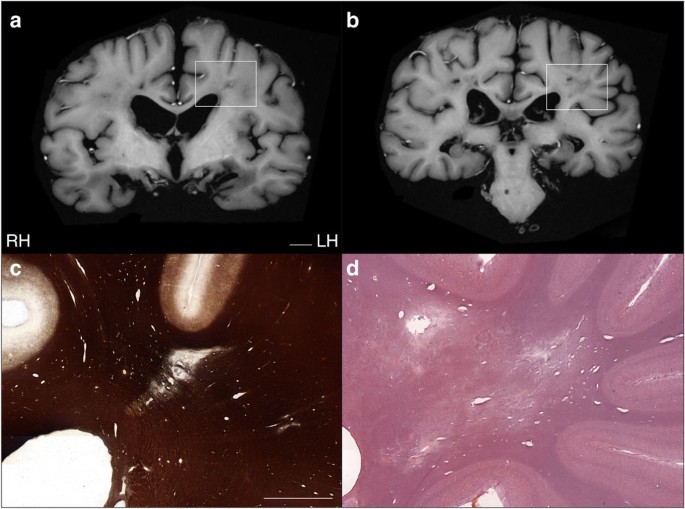
( a , b ) Lesions in the deep WM were visible in postmortem T1-weighted MRI images acquired ex situ (scale bar, 1 cm) and were confirmed by myelin silver-impregnation 40 , 46 ( c ) and haematoxylin and eosin (H&E) staining ( d ). Scale bar, 5 mm.
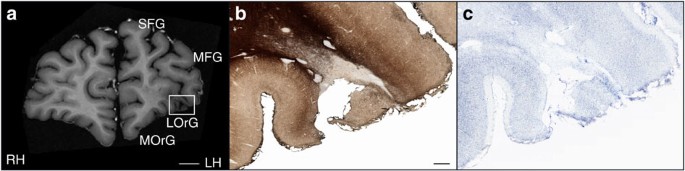
The reconstructed anatomical volume revealed a small lesion in the lateral orbital gyrus of the left frontal lobe. This is the only lesion affecting the gross structure of the cerebral cortex outside of the MTL. ( a ) T1-weighted MRI of the fixed brain acquired ex situ before cryo-sectioning. Scale bar, 1 cm. The same lesion is shown in histological material that was stained for myelinated fibres 40 , 46 ( b ) and neuronal cell bodies ( c ). Scale bar, 1 mm.
The 3D microscopic model of H.M.’s brain contained clues that help understand the surgery performed in 1953. Scoville approached the MTL of both hemispheres through two small trephine holes ( ∼ 3.8 cm in diameter) drilled above the orbits 17 . The ablation of the tip of the temporal lobe, the uncus and the amygdala was made with a scalpel; the sharp edge of the resections is noticeable in the anatomical and histological images ( Fig. 2d–f ). The more posterior MTL tissue was removed by suction; the fact that the periventricular portion of the hippocampus escaped the ablation can be explained by tracing the trajectory of the suction tube. Based on the reconstructed volume, it is clear that Scoville reached beyond and below the posterior hippocampus as indicated by the position of the surgical clip in the sagittal view of each hemisphere ( Fig. 3 ), although the placement of the clips was also contingent on the pattern of the surface vasculature.
We could reliably identify only a very minimal amount of EC in H.M.’s brain based on cytoarchitecture. The EC (via its superficial layers) is the gateway to the hippocampus for the inflow of information from the cerebral cortex and subcortical nuclei. The hippocampus has reciprocal connections to neocortical areas that deliver the original input via the deep layers of the EC and parahippocampal gyrus, creating a loop that supports the consolidation of long-term memories. Our study confirmed that this circuit was severely compromised by the ablation.
The hippocampus and EC also connect directly (that is, monosynaptically) to specific brain regions such as the nucleus accumbens, amygdala, cingulate cortex and orbitofrontal cortex. Input to and from the hippocampus is arranged along its anatomical length (as defined in this communication) according to an anterior-to-posterior functional gradient. For example, the amygdala is connected to anterior sections of the EC and hippocampus, while the visual cortex (largely residing in the occipital, parietal, lateral and ventral temporal lobes) is functionally linked to the posterior hippocampus. This organization is also reflected in the topography of differential gene expression and specific functional activation 33 , 34 , 35 . According to these maps, and based on the gradient of connectivity within the MTL and with other brain structures, the hippocampus appears broadly subdivided into an anterior segment that supports emotion, stress and sensorimotor integration, and a posterior part dedicated to declarative memory and neocortex-supported cognition 36 , 37 . According to this model, the almost complete removal of the EC in both hemispheres, resulting in severe disconnection of the remaining hippocampus, would have made a more significant contribution to H.M.’s declarative memory impairment than the ablation of the anterior hippocampus.
The excision of the anterior hippocampus, together with the bulk of the amygdala, may explain H.M.’s dampened expression of emotions, poor motivation and lack of initiative 19 . The fact that he was impaired in reporting internal states such as pain, hunger and thirst and his apparent lack of initiative was ascribed to the almost complete removal of the amygdala, although it remains difficult to distinguish between direct injury to the amygdala and disruption of connections into and out of this complex structure 6 , 38 . The sparing of the posterior segment of the parahippocampal cortex may validate observations of H.M.’s preserved visual perception, perceptual learning and perceptual priming 6 , 39 . A detailed architectonic survey of occipital, parietal and ventral temporal areas 40 , 41 could provide new clues about the neural circuits H.M. engaged during the performance of numerous perceptual tasks.
Histological staining revealed neuronal integrity in the CA4 field of the remaining hippocampus 42 and a normal compact pattern of the granule cell layer of the dentate gyrus ( Fig. 5 ). These findings raise interesting questions regarding the functional viability of H.M.’s remaining hippocampal tissue because according to established models of hippocampal connectivity, the gap in the MTL created by the surgery would have interrupted crucial connections to and from the posterior hippocampus. Additional quantitative analyses involving unbiased stereological methods can provide information regarding neuronal size and number 43 in this region. Combined with molecular approaches such as antibody staining to assess synaptic density, patterns of gliosis, and the integrity of afferent and efferent fibres, these studies could contribute further insight on the intrinsic and long-range connectivity of the human hippocampus.
The lesion on the left frontal lobe was circumscribed to a small area within the left lateral orbital gyrus ( Fig. 1 c), and it involved the cortical ribbon as well as the underlying WM ( Fig. 7 ). Because of the removal of all leptomeninges as part of the preparation of the brain prior to sectioning, it is not possible to determine the status of the immediate subpial zone in the area of the lesion, which would have assisted in discriminating a mechanical laceration from a vascular injury. As mentioned above, Scoville performed the removal of deep MTL structures while each frontal lobe was retracted to provide access to the deeper temporal structures; thus, judging from the orientation of the ablation with respect to the position of the trephine holes, the scar in the left prefrontal cortex could plausibly have been created at the time of the surgery.
In the course of the initial fixation, when the specimen was suspended upside down, the damaged portion of the temporal lobes collapsed; this geometrical artefact of fixation, combined with the relative shrinkage of the whole specimen during histological processing, should be considered when our resultsare compared with previous MRI scans obtained in vivo . It should be noted, however, that fixation, combined with cryoprotection, produced only an estimated 5–7% reduction in volume of the whole brain and these gross changes did not affect the morphology of the spared hippocampus.
Attempts to provide structure–function correlations based on the postmortem evaluation of H.M.’s MTL require a parallel assessment of age-related neuropathologic processes that developed progressively in his brain. MRI demonstrated extensive WM T2-hyperintensities, which were not related to the surgery; these were confirmed in histological sections ( Fig. 6 ). Although H.M. suffered a minor head injury before age 10, this kind of event does not typically produce long-lasting traumatic injuries that can be subsequently detected radiologically, especially in the absence of clinical signs. Rather, the nature and distribution of observed WM pathology suggest the manifestation of microvascular disease associated with hypertension. Diagnostic neuropathologic evaluation is still required to determine the types and burden of abnormalities and disease processes that occurred independently of the surgery as a result of aging and related to other aetiologies.
The purity and severity of H.M.’s memory impairment, combined with his willingness to participate in testing, made his case study uniquely influential in the field of memory research, as reflected in the broad range of scientific publications built on the studies to which he contributed 19 . During life, H.M. was the best-known and possibly the most studied patient in modern neuroscience; the availability of a large and organized collection of slices and digital anatomical images through the whole brain provides an unprecedented opportunity for collaborative and retrospective studies to continue 22 , 44 . The archive of images will also constitute a permanent digital trace for the case that defined two generations of memory research and that will likely remain as emblematic for neuroscience in the future as it is today.
Ethics statement
The study was reviewed and approved by one of UC San Diego’s Institutional Review Boards in accordance with the requirements of the Code of Federal Regulations for the Protections of Human Subjects. The removal and preservation of H.M.’s brain at autopsy, as well as disclosure of protected health information about him, was authorized by his legally appointed representative.
Preparation of the brain
Shortly after his death, H.M’s body was transported to the Anthinoula A. Martinos Center for Biological Imaging, in Charlestown, MA, where 9 h of in situ scanning was performed 21 . Following this imaging session, an autopsy was conducted; the brain removed, weighed (the total brain weight was 1,300 g) and then fixed in standard buffered formalin (4% formaldehyde; postmortem interval of ∼ 14 h). The brain was fixed for 10 weeks at 4 °C with three changes of fixative during that time; it was suspended upside down, hung by the basilar artery. When the tissue was firm enough, the brain was immersed in fixative laying on a cushion of hydrophilic cotton. Subsequently, multiple series of MRI scans of the fixed specimen were acquired in 3T and 7T scanners 21 .
H.M.’s brain was transported to The Brain Observatory in February 2009, where fixation in formaldehyde continued for 2 more months. After this additional period of fixation, the brain was imaged in a 1.5 T General Electric (GE) Excite MRI scanner using an eight-channel transmit-receive head coil ( Figs 6a,b and 7a ). We used a T1-weighted, 3-D Inversion-Recovery Fast Gradient Echo (IR-FSPRG) sequence (image matrix: 512 × 512; Slice thickness: 1mm; FOV: 256; TE: 15; 18 excitations, or NEX. Duration of the scan: ∼ 7 hours). During the scan the brain was soaked in formaldehyde solution and contained in a watertight Plexigas chamber designed to fit inside the coil above mentioned. Following MRI, the brain was immersed in solutions of increasing concentration of sucrose; specifically, 10, 20 and 30% in 4% formaldehyde solution, phosphate-buffered to pH 7.4. Treatment with sucrose lasted ∼ 10 months: it was meant to minimize the formation of ice crystals and reduced the risk of creating cracks when the brain was frozen.
Following fixation and cryoprotection, the leptomeninges were removed and the brain was embedded in a rectangular cast of 10% gelatin. The whole-brain-gelatin block was chilled to −40 °C by immersion in recirculating isopentane (2-methylbutane) using a custom-built insulated chamber. As the brain froze, the temperature of the solvent was constantly monitored and recorded via a probe connected to a personal computer running a custom programme (Labview, National Instruments Inc. Austin, TX, USA). The brain was thoroughly frozen after ∼ 5 h, when the temperature of the block and that of the surrounding fluid reached a stable equilibrium, based on probe data. The frozen block was firmly attached to the stage of a heavy-duty sliding microtome (Polycut SM 2500; Leica Microsystems Inc., Buffalo Grove, IL) and kept frozen by an assembly of interlocking hollow cuffs that transferred heat through a continuous flow of cold ethanol; the alcohol was recirculated and maintained at −40 °C by a battery of industrial chillers.
A second apparatus was mounted to the microtome to support a digital camera and lighting system directly above the microtome stage; it was designed to acquire high-resolution images of the surface of the block (gelatin and brain) through the entire slicing procedure. These images were the basis for 3D reconstruction and currently serve as a digital catalogue representing the collection of tissue slices.
Image acquisition and volume reconstruction
Tomographic images were acquired using a digital single-lens reflex camera (Nikon D700, Nikon Inc., Melville, NY) bearing a 35-mm lens (AF NIKKOR 35 mm f/2D), which was connected to a PC workstation and controlled remotely. A pair of opposite, light-emitting diode arrays were pointed at the surface of the block at an angle of 45°, providing constant illumination during the cutting procedure. The image acquisition sequence was fully automated; exposure was triggered before each new stroke was initiated. Image files were saved directly on disk in raw (uncompressed) format measuring 4,256 × 2,832 pixels (equivalent to a resolution of 46 μm per pixel). The microtome camera assembly was designed to trigger each exposure every time the microtome stage stopped at a fixed position so that the resulting image stack was registered mechanically. Dedicated team members maintained a log detailing file acquisition and inspected every newly acquired image in real time. Later, we used the quality control report to identify image artifacts in the series so that ad hoc post-processing routines for 3D reconstruction could be applied.
A Canon XLH1A digital HD camcorder (Canon U.S.A., Inc. Lake Success, NY) was used to record the procedure and provide a live feed of a close up of the brain within the microtome assembly on the web. Two additional views, of the microtome console and a wide-angle view of the lab, were provided via Microsoft LifeCam Cinema webcams (Microsoft Inc. Redmond, WA). The video from each of the three views was converted to flash with Adobe Flash Media Live Encoder 3.1 and streamed using Adobe Flash Media Server 3.5 (Adobe Systems Incorporated, San Jose, CA, USA).
Images acquired during the cutting procedure were transferred to a Dell PowerEdge 7500 workstation running a Windows 7, 64-bit operating system with 192 GB of DDR3 dynamic random-access memory (DRAM). The digital 3D volume representing the whole brain was assembled using AMIRA. Scaling factors were derived from the in-plane resolution of the images (converted from pixels to mm) and the slicing interval (that is, section thickness). The resolution of the data set was 46 × 46 × 70μm and occupied ∼ 56 GB on disk. Access to large amounts of DRAM allowed for the efficient manipulation and inspection of the entire anatomical volume. Measurements were conducted on a volume created from images that were down-sampled to 2,550 × 2,550 pixels (voxel dimensions were x : 56.9 μm; y : 56.9 μm and z : 70 μm).
The images were cropped to the edge of the gelatin block and corrected for any changes in brightness produced by fluctuations in ambient lighting that occurred during the 3-day procedure. As the ratio between brain tissue and gelatin matrix varied considerably at different levels of the series of anatomical images, we created a mask for brain tissue only and used it to adjust levels across the series. This process produced a uniform volumetric data set that was consistent in terms of luminosity and contrast in any arbitrary plane. Close inspection of the final 3D reconstruction identified occasional shifts in alignment, most likely due to accidental mechanical shifts in the equipment. Consequently, the position of outlier images was corrected by applying 2D rigid transformations (translation and rotation). The transformation matrix was computed with a local search algorithm that used a least-squares metric to compare intensities between each pair of consecutive images.
3D measurements of the hippocampus
The delineation of hippocampal structures was performed on the 3D reconstruction created from the series of digital images acquired in the course of the slicing procedure. Three main orthogonal planes were visualized concurrently in the segmentation editor of AMIRA to ensure accuracy. In the coronal plane, the hippocampus was identified starting at the posterior-most level of the mammillary bodies and the structure withered at the level of the inferior colliculi. The delineation of the inferior medial border (that is, the border between the subiculum and parahippocampal cortex) was aided by the identification of the perforant pathway in the anatomical images and changes in cytoarchitecture in corresponding Nissl-stained sections. Sagittal views afforded a clear demarcation of the hippocampus’ posterior border ( Fig. 3 ); the alveus was included in the calculation of the volume 45 . The volume of the hippocampus in each hemisphere was calculated as the number of tissue voxels comprised in the delineations multiplied by the individual voxel dimensions in mm.
The anatomical central axis of the hippocampus was defined as a 3D curve fit with a given stiffness along a path through the field of labelled voxels. We calculated a straight-line path using principal component analysis of a binary segmentation of the region of interest. The 3D line was sampled at uniform intervals (10 voxels) to define control points. The first and last control points of the line were set to the 3D locations defined by the furthermost extent of the object along the line. To improve the location of the control points, we sectioned the object at positions halfway between each pair of control points, using a plane that was orthogonal to the curve’s tangent direction at that location, and yielding one section for each control point. For each section of the binary object, we calculated its centre of mass and moved the corresponding control point towards that location. After the adjustments of all control points, a curve-smoothing operation was executed that used the predefined stiffness constraint on the curve. The extent of smoothing controlled the stiffness attached to the curve. The sectioning, adjustment and smoothing steps were repeated until convergence was achieved. This expectation–maximization approach iteratively adjusted the location of the curve’s control points towards a solution that intersected the object uniformly and had a given stiffness. The resulting 3D curve defined the ‘geodesic’ axis of the region of interest, and it was the basis for the actual anatomical length measurement.
Authors contributions
J.A. designed the neuroanatomical study and major instrumentation. M.P.F. conducted the brain extraction at autopsy assisted by J.A. Serial sectioning and tomographic imaging of the brain specimen was performed by J.A. with the assistance of N.M.S., P.M., N.T., J.K., A.G. and N.B., H.B., N.M.S., P.M. and J.A. developed the 3D model of the brain from cross-sectional image data acquired during the dissection. H.B. developed the 3D measurement and image registration tools. J.A., P.M. and C.S produced the stained histological slides. J.A. wrote the manuscript with significant editing contributions by S.C. and M.P.F. Photographs and figures were created by J.A. and C.S. Additional background research was conducted by R.K.
Additional information
How to cite this article: Annese, J. et al. Postmortem examination of patient H.M.’s brain based on histological sectioning and digital 3D reconstruction. Nat. Commun. 5:3122 doi: 10.1038/ncomms4122 (2014).
Scoville, W. B. & Milner, B. Loss of recent memory after bilateral hippocampal lesions. J. Neurol. Neurosurg. Psychiatr. 296 , 1–22 (1957).
Google Scholar
Corkin, S. Lasting consequences of bilateral medial temporal lobectomy: clinical course and experimental findings in H.M. Sem. Neurol. 4 , 249–259 (1984).
Article Google Scholar
Corkin, S. Tactually-guided maze learning in man: Effects of unilateral cortical excisions and bilateral hippocampal lesions. Neuropsychologia 3 , 339–351 (1965).
Corkin, S. Acquisition of motor skill after bilateral medial temporal-lobe excision. Neuropsychologia 6 , 255–265 (1968).
Woodruff-Pak, D. S. Delay and trace paradigms. Behav. Neurosci. 107 , 911–925 (1993).
Article CAS Google Scholar
Milner, B., Corkin, S. & Teuber, H.-L. Further analysis of the hippocampal amnesiac syndrome: 14-year follow-up study of H.M. Neuropsychologia 6 , 215–234 (1968).
Keane, M. M., Gabrieli, J. D. E., Mapstone, H. C., Johnson, K. A. & Corkin, S. Double dissociation of memory capacities after bilateral occipital-lobe or medial temporal-lobe lesions. Brain 118 , 1129–1148 (1995).
Milner, B. Memory and the medial temporal regions of the brain. In Biology of Memory (eds Pribram K. H., Broadbent D. E. )29–50Academic Press (1970).
Milner, B. The medial temporal lobe amnesic syndrome. Psychiatr. Clin. N. Am. 28 , 599–611 (2005).
Cohen, N. J. & Squire, L. R. Preserved learning and retention of pattern analyzing skill in amnesia: dissociation of knowing how and knowing that. Science 210 , 207–209 (1980).
Article CAS ADS Google Scholar
Steinvorth, S., Levine, B. & Corkin, S. Medial temporal lobe structures are needed to re-experience remote autobiographical memories: evidence from H.M. and W.R. Neuropsychologia 43 , 479–496 (2005).
Squire, L. R. & Wixted, J. T. The cognitive neuroscience of human memory since H.M. Ann. Rev. Neurosci. 34 , 259–288 (2011).
Tulving, E. Episodic and semantic memory. In: Organization of Memory (eds Tulving E., Donaldson W. )381–402Academic Press (1972).
Gabrieli, J. D. E., Cohen, N. J. & Corkin, S. The impaired learning of semantic knowledge following bilateral medial temporal-lobe resection. Brain Cogn. 7 , 157–177 (1988).
O’Kane, G., Kensinger, E. A. & Corkin, S. Evidence for semantic learning in profound amnesia: an investigation with patient H.M. Hippocampus 14 , 417–425 (2004).
Scoville, W. B. The limbic lobe in man. J. Neurosurg. 11 , 64–66 (1954).
Scoville, W. B., Dunsmore, R. H., Liberson, W. T., Henry, C. E. & Pepe, A. Observations on medial temporal lobotomy and uncotomy in the treatment of psychotic states. Res. Pub. Assoc. Res. Nerv. Ment. Dis. 31 , 347–373 (1953).
CAS Google Scholar
Scoville, W. B. Amnesia after bilateral mesial temporal-lobe excision: Introduction to case H.M. Neuropsychologia 6 , 211–213 (1968).
Corkin, S., Amaral, D. G., González, R., Johnson, K. & Hyman, B. T. H.M.’s medial temporal lobe lesion: findings from magnetic resonance imaging. J. Neurosci. 17 , 3964–3979 (1997).
Salat, D. H. et al. Neuroimaging H.M.: a 10-year follow-up examination. Hippocampus 16 , 936–945 (2006).
van der Kouwe, A. et al. Technical description of post-mortem magnetic resonance neuroimaging of H.M. Program No. 714.17. 2010 Neuroscience Meeting Planner. San Diego, CA: Society for Neuroscience, 2010. Online.
Annese, J. Deconstructing Henry: the neuroanatomy and neuroinformatics of the brain of the amnesic patient H.M. Program No. 397.18. 2010 Neuroscience Meeting Planner. San Diego, CA: Society for Neuroscience, 2010. Online.
Talairach, J. & Tournoux, P. Co-Planar Stereotaxic Atlas of the Human Brain Thieme Medical Publishers (1988).
Wilson, B. A., Baddeley, A. D. & Kapur, N. Dense amnesia in a professional musician following herpes simplex virus encephalitis. J. Clin. Exp. Neuropsychol. 17 , 668–681 (1995).
Stefanacci, L., Buffalo, E. A., Schmolck, H. & Squire, L. R. Profound amnesia after damage to the medial temporal lobe: a neuroanatomical and neuropsychological profile of patient E.P. J. Neurosci. 20 , 7024–7036 (2000).
Insausti, R., Annese, J., Amaral, D. G. & Squire, L. R. Human amnesia and the medial temporal lobe illuminated by neuropsychological and neurohistological findings for patient E.P. Proc. Natl Acad. Sci. USA 110 , E1953–E1962 (2013).
Damasio, A. R., Eslinger, P., Damasio, H., van Hoesen, G. W. & Cornell, S. Multimodal amnesic syndrome following bilateral and basal forebrain damage. Arch. Neurol. 42 , 252–259 (1985).
Annese, J. The importance of combining MRI and large-scale digital histology in neuroimaging studies of brain connectivity and disease. Front. Neuroinform. 6 , 1–5 (2012).
Buchen, L. Famous brain set to go under the knife. Nature 462 , 403 (2009).
Insausti, R. et al. MR volumetric analysis of the human entorhinal, perirhinal, and temporopolar cortices. Am. J. Neuroradiol. 19 , 659–671 (1998).
CAS PubMed Google Scholar
De Marcos, F. A., Ghizoni, E., Kobayashi, E., Li, L. M. & Cendes, F. Cerebellar volume and long-term use of phenytoin. Seizure 12 , 312–315 (2003).
Ghatak, N. R., Santoso, R. A. & McKinney, W. M. Cerebellar degeneration following long-term phenytoin therapy. Neurology 26 , 818–820 (1976).
Zhao, X. et al. Transcriptional profiling reveals strict boundaries between hippocampal subregions. J. Comp. Neurol. 441 , 187–196 (2001).
Thompson, C. et al. Genomic anatomy of the hippocampus. Neuron 60 , 1010–1021 (2008).
Fanselow, M. & Dong, H. Are the dorsal and ventral hippocampus functionally distinct structures? Neuron 65 , 7–19 (2010).
Small, S., Nava, A., DeLaPaz, R., Mayeux, R. & Stern, Y. Circuit mechanisms underlying memory encoding and retrieval in the long axis of the hippocampal formation. Nat. Neurosci. 4 , 442–449 (2001).
Lavenex, P. & Amaral, D. Hippocampal-neocortical interaction: a hierarchy of associativity. Hippocampus 10 , 420–430 (2000).
Hebben, N., Corkin, S., Eichenbaum, H. & Shedlack, K. Diminished ability to interpret and report internal states after bilateral medial temporal resection: case H.M. Behav. Neurosci. 99 , 1031–1039 (1985).
Corkin, S. What’s new with the amnesic patient H.M.? Nat. Rev. Neurosci. 3 , 153–160 (2002).
Annese, J., Pitiot, A., Dinov, I. & Toga, A. A myeloarchitectonic method for the structural classification of cortical areas. NeuroImage 21 , 15–26 (2004).
Annese, J., Gazzaniga, M. & Toga, A. Localization of the human cortical visual area MT based on computer-aided histological analysis. J. Neurosci. 15 , 1044–1053 (2005).
Duvernoy, H. M. The Human Hippocampus: Functional Anatomy, Vascularization and Serial Sections with MRI, Third Edn Springer (2005).
West, M. J. & Gundersen, H. J. Unbiased stereological estimation of the number of neurons in the human hippocampus. J. Comp. Neurol. 296 , 1–22 (1990).
Annese, J. From the jar to the world wide web: designing a public digital library for the human brain. Interdiscipl. Sci. Rev. 38 , 222–231 (2013).
Insausti, R. & Amaral, D. G. Hippocampal formation. In The Human Nervous System 2nd edn (eds Paxinos G., Mai J. K. )871–914Academic Press (2004).
Gallyas, F. Silver staining of myelin by means of physical development. Neurol. Res. 1 , 203–209 (1979).
Download references
Acknowledgements
The work was supported by grants from the National Science Foundation (NSF—SGER 0714660, J.A., Principal Investigator), the Dana Foundation (Brain and Immuno-Imaging Award, 2007-4234, J.A., Principal Investigator) and by private contributions from viewers of the web broadcast of the dissection. In the course of the study, Dr. Annese was in part supported by two research grants from the National Eye Institute, R01 EY018359–02 and ARRA R01 EY018359–02S1 (J.A., Principal Investigator) and the National Institute of Mental Health, R01MH084756 (J.A., Principal Investigator). The authors would like to thank Herbert and Sharon Lurie, M.S., for their generous contribution towards publishing the article on an open access basis. We would like to dedicate the project and this publication to H.M. He transformed his difficult experience into a lifelong contribution to scientific research on the mechanisms supporting human memory. This study also honours all the researchers who conducted studies with H.M. when he was alive, and Brenda Milner, in particular, who first demonstrated the importance of his case. The authors would like to acknowledge the critical contribution of David Malmberg in the development of the instrumentation and thank Dr. Elizabeth Murphy for editorial assistance.
Author information
Natasha Thomas, Junya Kayano and Alexander Ghatan: These authors contributed equally to this work
Authors and Affiliations
The Brain Observatory, San Diego, 92101, California, USA
Jacopo Annese, Natalie M. Schenker-Ahmed, Hauke Bartsch, Paul Maechler, Colleen Sheh, Natasha Thomas, Junya Kayano, Alexander Ghatan, Noah Bresler & Ruth Klaming
Department of Radiology, University of California San Diego, San Diego, 92093, California, USA
Jacopo Annese, Natalie M. Schenker-Ahmed, Hauke Bartsch, Paul Maechler, Colleen Sheh & Ruth Klaming
C.S. Kubik Laboratory for Neuropathology, Massachusetts General Hospital and Harvard Medical School, 02114, Massachusetts, USA
Matthew P. Frosch
Department of Brain and Cognitive Sciences, Massachusetts Institute of Technology, 02139, Massachusetts, USA
Suzanne Corkin
You can also search for this author in PubMed Google Scholar
Corresponding author
Correspondence to Jacopo Annese .
Ethics declarations
Competing interests.
The authors declare no competing financial interests.
Rights and permissions
This work is licensed under a Creative Commons Attribution-NonCommercial-NoDerivs 3.0 Unported License. To view a copy of this license, visit http://creativecommons.org/licenses/by-nc-nd/3.0/
Reprints and permissions
About this article
Cite this article.
Annese, J., Schenker-Ahmed, N., Bartsch, H. et al. Postmortem examination of patient H.M.’s brain based on histological sectioning and digital 3D reconstruction. Nat Commun 5 , 3122 (2014). https://doi.org/10.1038/ncomms4122
Download citation
Received : 13 October 2013
Accepted : 16 December 2013
Published : 28 January 2014
DOI : https://doi.org/10.1038/ncomms4122
Share this article
Anyone you share the following link with will be able to read this content:
Sorry, a shareable link is not currently available for this article.
Provided by the Springer Nature SharedIt content-sharing initiative
This article is cited by
Immunohistochemical field parcellation of the human hippocampus along its antero-posterior axis.
- Emilio González-Arnay
- Isabel Pérez-Santos
- Carmen Cavada
Brain Structure and Function (2024)
High Pressure Hosing-Drone Dynamics and Controls
- Blake Hament
Journal of Intelligent & Robotic Systems (2023)
Convolutional neuronal networks combined with X-ray phase-contrast imaging for a fast and observer-independent discrimination of cartilage and liver diseases stages
- Johannes Stroebel
- Annie Horng
Scientific Reports (2020)
Pavlovian patterns in the amygdala
- Bruno B. Averbeck
Nature Neuroscience (2019)
Dissecting the pathobiology of altered MRI signal in amyotrophic lateral sclerosis: A post mortem whole brain sampling strategy for the integration of ultra-high-field MRI and quantitative neuropathology
- Menuka Pallebage-Gamarallage
- Sean Foxley
- Olaf Ansorge
BMC Neuroscience (2018)
By submitting a comment you agree to abide by our Terms and Community Guidelines . If you find something abusive or that does not comply with our terms or guidelines please flag it as inappropriate.
Quick links
- Explore articles by subject
- Guide to authors
- Editorial policies
Sign up for the Nature Briefing newsletter — what matters in science, free to your inbox daily.

- Skip to main content
- Keyboard shortcuts for audio player
Research News
H.m.'s brain and the history of memory.
Brian Newhouse
In 1953, radical brain surgery was used on a patient with severe epilepsy. The operation on "H.M." worked, but left him with almost no long-term memory. H.M. is now in his 80s. His case has helped scientists understand much more about the brain.
Web Resources
SCOTT SIMON, host:
Fifty years ago this month, the Journal of Neurology, Neurosurgery and Psychiatry published the findings of a remarkable case. A young man who had undergone an experimental brain operation had lost his ability to retain new memories. He could remember things from his life before the operation but any new face or fact, he completely forgot within minutes. Researchers at that time studied him. And it turns out their discoveries opened the modern era of memory research, what's involved every time we say I remember. That young man is now in his 80s. And as Brian Newhouse reports, scientists are still learning from him.
(Soundbite of crickets)
BRIAN NEWHOUSE: If you close your eyes and just listen for a moment, you may find yourself going somewhere, back in memory. Back maybe to a farm or a park or a lake. Other sounds may make those memories sharpen or change. Add still more and you may start to see particular faces or even smell wood smoke. Remember?
(Soundbite of laughter)
NEWHOUSE: This is the power of memory, the system that captures pictures, smells, sounds, events, directions - endless amounts of information every day and then seconds or decades later calls it up for us. Memories - what we've learned and what we've done - in a large sense make us who we are. To appreciate this, think if your ability to form any new memories were suddenly cut off.
Who would you be? By studying people who've lost their memories, scientists have learned enormous amounts about how learning and memory work in healthy brains. And what they used to think was relatively straightforward they've since found it's fascinatingly complex, thanks in large part to one man.
He's the most famous patient in the study of the human brain today. He's written up in textbooks and dozens of scientific papers. The modern era of memory research essentially began with him, yet very few people know his name or have ever seen him, despite the efforts of journalists, filmmakers and TV networks, all of whom have asked to photograph, film or interview him. Outside the circle of his family and caregivers, he's known only by his initials, H.M. His guardians recently agreed to release audio recordings made of him in the early 1990s talking to scientists. This is the first time a wide audience has been able to hear his voice.
Dr. BRENDA MILNER (McGill University) : When you're not at MIT, what do you do during a typical day?
H.M. (Patient): See, that's what I don't - I don't remember things.
Dr. MILNER: Uh-huh.
H.M. was a very pleasant normal young man, but he had suffered from very severe epilepsy all his life, really. It made him unable to hold down his job as an assembly worker. It made him very late in finishing high school, although he was quite intelligent.
NEWHOUSE: Brenda Milner is a British-born neuroscientist at McGill University in Montreal who first met H.M. in the mid-1950s.
Dr. MILNER: He had huge major and minor seizures, you know, huge convulsions, and also many, many lapses of consciousness every few minutes. He was in a very, very hopeless condition with his epilepsy.
NEWHOUSE: Dr. Milner came to know H.M. after Connecticut neurosurgeon William Beecher Scoville performed an experimental operation to help relieve H.M.'s seizures. Dr. Scoville thought if he could remove the part of H.M.'s brain where the seizures originated, it might stop them.
Dr. MILNER: And this operation was carried out when - in '53, 1953, September - when H.M. was 27. The operation did have an enormously beneficial effect on the epilepsy so that H.M. has maybe now one big seizure a year, and so the clinical hunch about the epilepsy was justified. But at obviously a horrendous price.
(Soundbite of piano)
Dr. MILNER: Who is the president of the United States now?
H.M.: That I don't - I couldn't tell you. I don't remember exactly at all.
Dr. MILNER: Is it a man or a woman?
H.M.: I think it's a man.
Dr. MILNER: His initials are G.B. Does that help?
H.M.: No, it doesn't help.
NEWHOUSE: The horrendous price that H.M. paid was a severe case of amnesia. Not the amnesia of Hollywood, where a person forgets everything about his past, but in H.M. it's his ability to acquire new memories, to commit to memory even the simplest events of his day or the world around him, and then to effectively retrieve those memories. Put a finger above your ear. If you were able to push that finger into your head about two inches, you'd be in the area called the medial temporal lobe. There's one on each side of the brain. In the 1950s, Dr. Scoville theorized that these were the general areas involved in H.M.'s epilepsy. But in trying to alleviate H.M.'s seizures, Dr. Scoville removed most of the medial temporal lobes, including much of the hippocampus. This unintentional experiment showed that the hippocampus and medial temporal lobes are where the brain converts short term memory into long term memory.
Dr. MILNER: Do you know what you did yesterday?
H.M.: No, I don't.
Dr. MILNER: How about this morning?
H.M.: I don't even remember that.
Dr. MILNER: Could you tell me what you had for lunch today?
H.M.: I don't know, to tell you the truth.
NEWHOUSE: H.M.'s condition has also helped scientists understand how and where the brain processes different types of memory. Scientists now know that some brain structures are involved in things like phone numbers we keep only for a few seconds, while others deal with the day's appointments. And still others determine which childhood experiences will stay with us until we die. Now, when you can't remember what you did yesterday or had for lunch today, how do you build a life? Unfortunately, there's been no silver bullet for H.M. Holding a job or even having friends, normal things for most of us with working memories, have been beyond him. H.M. is now in his early 80s and living in a Connecticut nursing home. And he is still what doctor's call profoundly amnesic.
Dr. Brenda Milner studied and tested H.M.'s memory for years after his surgery. In the early 1960's she asked Suzanne Corkin, a young American neuroscientist working in her lab, to help. Dr. Corkin, now at MIT in Cambridge, Massachusetts, has interview H.M. many times since then.
Dr. SUZANNE CORKIN (MIT): He is in my PhD thesis and I have followed his progress for the last 43 years. And he still doesn't know who I am.
NEWHOUSE: Despite H.M.'s difficulties with creating new memories, his old ones from his childhood are intact, especially about major world events.
Dr. MILNER: What happened in 1929?
H.M.: The stock market crashed.
Dr. MILNER: It sure did.
NEWHOUSE: H.M.'s clear memory of events before his surgery showed that although the hippocampus was necessary to make new long term memories, it wasn't needed to retrieve old ones. In the mid-1950's the surgeon Dr. Scoville was mortified to discover that his operation had ruined so much of H.M.'s memory, even though it did relieve H.M.'s epilepsy and probably saved his life. Afterward, Dr. Scoville campaigned widely against the procedure. So H.M. is what scientists call an N(ph) of one. He is the only patient whose had this operation. That makes H.M. unique in science today. But that's not the only thing, or even the most important. Again, Dr. Corkin.
Dr. CORKIN: One thing that still fascinates us today is the fact that in real life, in spite of his profound amnesia, he is able to learn a meager amount of semantic information, knowledge about public figures, people who became famous after his operation. The fact that he can remember anything at all is just enough to make the experimenter fall right off her chair.
Dr. MILNER: How about 1963? Someone was assassinated.
H.M.: He'd been a president.
Dr. MILNER: That's right.
H.M.: And he was assassinated.
Dr. MILNER: What was his name?
H.M.: He had been, like you said, he had been a president.
Dr. MILNER: His initials are JFK.
H.M.: Kennedy.
Dr. MILNER: That's right. What was his first name?
H.M.: John.
Dr. CORKIN: The other day, I was talking to a nurse in his nursing home, just asking her a few questions about him. And after we talked, she went into his room and she said, Oh, I was just talking to a friend of yours from Boston, Dr. Corkin. And H.M. said, Suzanne?
Dr. CORKIN: Now, this is really astonishing. Now, he doesn't know who I am. He doesn't know what I do or what my connection is with him. But he has learned to associate my first name and my last name. And that was another surprise for all of us.
NEWHOUSE: Somehow the man who couldn't form new memories had found a way to learn new things. It was a remarkable discovery that radically altered our understanding of how learning and memory work. Before H.M., doctors believed there was a single memory store through which all information moved and was processed, and that it all resided in one spot in the brain, what you might call a single address.
Now, based on what they've learned from H.M., doctors understand memory to be much more dynamic than that. They found that the brain has several different memory systems. We use what's called declarative memory any time we say I remember, and then recall that we had cereal for breakfast, or that the capital of Illinois is Springfield, or that these two notes on the piano are C and D.
NEWHOUSE: The other kind of memory is non-declarative. It's what we use to tie our shoes, ride a bike, or how to play the C-scale smoothly without thinking of the individual notes.
Again, Dr. Corkin.
Dr. CORKIN: We believe that when you remember something it's really an active process. You're not tuning into a few cells in your brain where a particular memory is stored. What you're really doing is creating a memory based on information that you have stored in many parts of your brain. Now, since H.M.'s operation, we know that there are multiple long term memory systems in the brain that have different addresses. I think his case inspired clinicians and scientists all over the world to find their H.M. and to make amazing discoveries. So it's sort of an ongoing adventure of the human mind and the human brain.
NEWHOUSE: Despite those discoveries, scientists admit they still don't know how it all works, how memories are culled from different parts of the brain and fused together. What they have learned, though, is that the brain's processes are far more intricate than they ever thought. And much of the credit for that goes to patient H.M. Even though H.M. can't look back over a lifetime of rich memories, his spirit seems untouched by that deficit in his brain.
Dr. CORKIN: What do you think you'll do tomorrow?
H.M.: Whatever is beneficial.
Dr. CORKIN: Good answer. Are you happy?
H.M.: Yes. Well, the way I figure it is, what they find out about me helps them to help other people.
NEWHOUSE: For NPR News, this is Brian Newhouse.
Copyright © 2007 NPR. All rights reserved. Visit our website terms of use and permissions pages at www.npr.org for further information.
NPR transcripts are created on a rush deadline by an NPR contractor. This text may not be in its final form and may be updated or revised in the future. Accuracy and availability may vary. The authoritative record of NPR’s programming is the audio record.
Hopkins Verbal Learning Test
- Reference work entry
- pp 1264–1265
- Cite this reference work entry
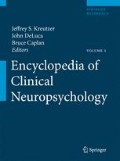
- Stacy Belkonen 5
6123 Accesses
7 Citations
This is a preview of subscription content, log in via an institution to check access.
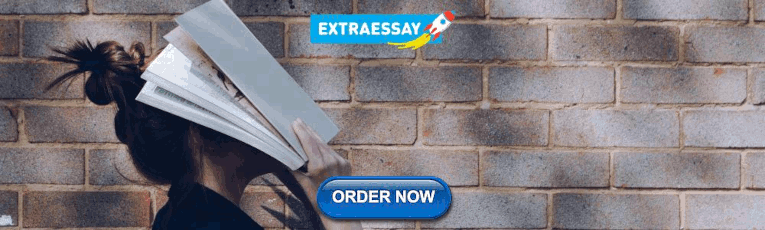
Access this chapter
- Available as PDF
- Read on any device
- Instant download
- Own it forever
- Available as EPUB and PDF
- Durable hardcover edition
- Dispatched in 3 to 5 business days
- Free shipping worldwide - see info
Tax calculation will be finalised at checkout
Purchases are for personal use only
Institutional subscriptions
References and Readings
Benedict, R. H. B., Schretlen, D., Groninger, L., & Brandt, J. (1998). The Hopkins verbal learning test-revised: Normative data and analysis of interform and test–retest reliability. Clinical Neuropsychologist, 12 , 43–55.
Google Scholar
Brandt, J. (1991). The Hopkins verbal learning test: Development of a new memory test with six equivalent forms. Clinical Neuropsychologist, 5 , 125–142.
Brandt, J., & Benedict, R. H. B. (2001). Hopkins verbal learning test – Revised. Administration manual . Lutz, FL: Psychological Assessment Resources.
Chiaravalloti, N. D., DeLuca, J., Moore, N. B., & Ricker, J. H. (2005). Treating learning impairments improves memory performance in multiple sclerosis: A randomized clinical trial. Multiple Sclerosis, 11 , 58–68.
PubMed Google Scholar
Rasmusson, D. X., Bylsma, F. W., & Brandt, J. (1995). Stability of performance on the Hopkins verbal learning test. Archives of Clinical Neuropsychology, 10 , 21–26.
Download references
Author information
Authors and affiliations.
Department of Rehab Medicine, Mount Sinai School of Medicine, 5 East 98th Street, 10029, New York, NY, USA
Stacy Belkonen
You can also search for this author in PubMed Google Scholar
Editor information
Editors and affiliations.
Physical Medicine and Rehabilitation, and Professor of Neurosurgery, and Psychiatry Virginia Commonwealth University – Medical Center Department of Physical Medicine and Rehabilitation, VCU, 980542, Richmond, Virginia, 23298-0542, USA
Jeffrey S. Kreutzer
Kessler Foundation Research Center, 1199 Pleasant Valley Way, West Orange, NJ, 07052, USA
John DeLuca
Professor of Physical Medicine and Rehabilitation, and Neurology and Neuroscience, University of Medicine and Dentistry of New Jersey – New Jersey Medical School, New Jersey, USA
Independent Practice, 564 M.O.B. East, 100 E. Lancaster Ave., Wynnewood, PA, 19096, USA
Bruce Caplan
Rights and permissions
Reprints and permissions
Copyright information
© 2011 Springer Science+Business Media, LLC
About this entry
Cite this entry.
Belkonen, S. (2011). Hopkins Verbal Learning Test. In: Kreutzer, J.S., DeLuca, J., Caplan, B. (eds) Encyclopedia of Clinical Neuropsychology. Springer, New York, NY. https://doi.org/10.1007/978-0-387-79948-3_1127
Download citation
DOI : https://doi.org/10.1007/978-0-387-79948-3_1127
Publisher Name : Springer, New York, NY
Print ISBN : 978-0-387-79947-6
Online ISBN : 978-0-387-79948-3
eBook Packages : Behavioral Science
Share this entry
Anyone you share the following link with will be able to read this content:
Sorry, a shareable link is not currently available for this article.
Provided by the Springer Nature SharedIt content-sharing initiative
- Publish with us
Policies and ethics
- Find a journal
- Track your research
- Share full article
Advertisement
Supported by
H. M., an Unforgettable Amnesiac, Dies at 82
By Benedict Carey
- Dec. 4, 2008
He knew his name. That much he could remember.
He knew that his father’s family came from Thibodaux, La., and his mother was from Ireland, and he knew about the 1929 stock market crash and World War II and life in the 1940s.
But he could remember almost nothing after that.
In 1953, he underwent an experimental brain operation in Hartford to correct a seizure disorder, only to emerge from it fundamentally and irreparably changed. He developed a syndrome neurologists call profound amnesia. He had lost the ability to form new memories.
For the next 55 years, each time he met a friend, each time he ate a meal, each time he walked in the woods, it was as if for the first time.
And for those five decades, he was recognized as the most important patient in the history of brain science. As a participant in hundreds of studies, he helped scientists understand the biology of learning, memory and physical dexterity, as well as the fragile nature of human identity.
On Tuesday evening at 5:05, Henry Gustav Molaison known worldwide only as H. M., to protect his privacy died of respiratory failure at a nursing home in Windsor Locks, Conn. His death was confirmed by Suzanne Corkin, a neuroscientist at the Massachusetts Institute of Technology, who had worked closely with him for decades. Henry Molaison was 82.
From the age of 27, when he embarked on a life as an object of intensive study, he lived with his parents, then with a relative and finally in an institution. His amnesia did not damage his intellect or radically change his personality. But he could not hold a job and lived, more so than any mystic, in the moment.
“Say it however you want,” said Dr. Thomas Carew, a neuroscientist at the University of California, Irvine, and president of the Society for Neuroscience. “What H. M. lost, we now know, was a critical part of his identity.”
At a time when neuroscience is growing exponentially, when students and money are pouring into laboratories around the world and researchers are mounting large-scale studies with powerful brain-imaging technology, it is easy to forget how rudimentary neuroscience was in the middle of the 20th century.
When Mr. Molaison, at 9 years old, banged his head hard after being hit by a bicycle rider in his neighborhood near Hartford, scientists had no way to see inside his brain. They had no rigorous understanding of how complex functions like memory or learning functioned biologically. They could not explain why the boy had developed severe seizures after the accident, or even whether the blow to the head had anything do to with it.
Eighteen years after that bicycle accident, Mr. Molaison arrived at the office of Dr. William Beecher Scoville, a neurosurgeon at Hartford Hospital. Mr. Molaison was blacking out frequently, had devastating convulsions and could no longer repair motors to earn a living.
After exhausting other treatments, Dr. Scoville decided to surgically remove two finger-shaped slivers of tissue from Mr. Molaison’s brain. The seizures abated, but the procedure especially cutting into the hippocampus, an area deep in the brain, about level with the ears left the patient radically changed.
Alarmed, Dr. Scoville consulted with a leading surgeon in Montreal, Dr. Wilder Penfield of McGill University, who with Dr. Brenda Milner, a psychologist, had reported on two other patients’ memory deficits.
Soon Dr. Milner began taking the night train down from Canada to visit Mr. Molaison in Hartford, giving him a variety of memory tests. It was a collaboration that would forever alter scientists’ understanding of learning and memory.
“He was a very gracious man, very patient, always willing to try these tasks I would give him,” Dr. Milner, a professor of cognitive neuroscience at the Montreal Neurological Institute and McGill University, said in a recent interview. “And yet every time I walked in the room, it was like we’d never met.”
At the time, many scientists believed that memory was widely distributed throughout the brain and not dependent on any one neural organ or region. Brain lesions, either from surgery or accidents, altered people’s memory in ways that were not easily predictable. Even as Dr. Milner published her results, many researchers attributed H. M.’s deficits to other factors, like general trauma from his seizures or some unrecognized damage.
“It was hard for people to believe that it was all due” to the excisions from the surgery, Dr. Milner said.
That began to change in 1962, when Dr. Milner presented a landmark study in which she and H. M. demonstrated that a part of his memory was fully intact. In a series of trials, she had Mr. Molaison try to trace a line between two outlines of a five-point star, one inside the other, while watching his hand and the star in a mirror. The task is difficult for anyone to master at first.
Every time H. M. performed the task, it struck him as an entirely new experience. He had no memory of doing it before. Yet with practice he became proficient. “At one point he said to me, after many of these trials, ‘Huh, this was easier than I thought it would be,’ ” Dr. Milner said.
The implications were enormous. Scientists saw that there were at least two systems in the brain for creating new memories. One, known as declarative memory, records names, faces and new experiences and stores them until they are consciously retrieved. This system depends on the function of medial temporal areas, particularly an organ called the hippocampus, now the object of intense study.
Another system, commonly known as motor learning, is subconscious and depends on other brain systems. This explains why people can jump on a bike after years away from one and take the thing for a ride, or why they can pick up a guitar that they have not played in years and still remember how to strum it.
Soon “everyone wanted an amnesic to study,” Dr. Milner said, and researchers began to map out still other dimensions of memory. They saw that H. M.’s short-term memory was fine; he could hold thoughts in his head for about 20 seconds. It was holding onto them without the hippocampus that was impossible.
“The study of H. M. by Brenda Milner stands as one of the great milestones in the history of modern neuroscience,” said Dr. Eric Kandel, a neuroscientist at Columbia University. “It opened the way for the study of the two memory systems in the brain, explicit and implicit, and provided the basis for everything that came later the study of human memory and its disorders.”
Living at his parents’ house, and later with a relative through the 1970s, Mr. Molaison helped with the shopping, mowed the lawn, raked leaves and relaxed in front of the television. He could navigate through a day attending to mundane details fixing a lunch, making his bed by drawing on what he could remember from his first 27 years.
He also somehow sensed from all the scientists, students and researchers parading through his life that he was contributing to a larger endeavor, though he was uncertain about the details, said Dr. Corkin, who met Mr. Molaison while studying in Dr. Milner’s laboratory and who continued to work with him until his death.
By the time he moved into a nursing home in 1980, at age 54, he had become known to Dr. Corkin’s M.I.T. team in the way that Polaroid snapshots in a photo album might sketch out a life but not reveal it whole.
H. M. could recount childhood scenes: Hiking the Mohawk Trail. A road trip with his parents. Target shooting in the woods near his house.
“Gist memories, we call them,” Dr. Corkin said. “He had the memories, but he couldn’t place them in time exactly; he couldn’t give you a narrative.”
He was nonetheless a self-conscious presence, as open to a good joke and as sensitive as anyone in the room. Once, a researcher visiting with Dr. Milner and H. M. turned to her and remarked how interesting a case this patient was.
“H. M. was standing right there,” Dr. Milner said, “and he kind of colored blushed, you know and mumbled how he didn’t think he was that interesting, and moved away.”
In the last years of his life, Mr. Molaison was, as always, open to visits from researchers, and Dr. Corkin said she checked on his health weekly. She also arranged for one last research program. On Tuesday, hours after Mr. Molaison’s death, scientists worked through the night taking exhaustive M.R.I. scans of his brain, data that will help tease apart precisely which areas of his temporal lobes were still intact and which were damaged, and how this pattern related to his memory.
Dr. Corkin arranged, too, to have his brain preserved for future study, in the same spirit that Einstein’s was, as an irreplaceable artifact of scientific history.
“He was like a family member,” said Dr. Corkin, who is at work on a book on H. M., titled “A Lifetime Without Memory.” “You’d think it would be impossible to have a relationship with someone who didn’t recognize you, but I did.”
In his way, Mr. Molaison did know his frequent visitor, she added: “He thought he knew me from high school.”
Henry Gustav Molaison, born on Feb. 26, 1926, left no survivors. He left a legacy in science that cannot be erased.

An official website of the United States government
The .gov means it’s official. Federal government websites often end in .gov or .mil. Before sharing sensitive information, make sure you’re on a federal government site.
The site is secure. The https:// ensures that you are connecting to the official website and that any information you provide is encrypted and transmitted securely.
- Publications
- Account settings
Preview improvements coming to the PMC website in October 2024. Learn More or Try it out now .
- Advanced Search
- Journal List
- HHS Author Manuscripts

H.M.’s Contributions to Neuroscience: A Review and Autopsy Studies
Jean c. augustinack.
1 Department of Radiology, Athinoula A. Martinos Center for Biomedical Imaging, Massachusetts General Hospital, Charlestown, Massachusetts
André J.W. van der Kouwe
David h. salat, thomas benner, allison a. stevens, jacopo annese.
2 The Brain Observatory, San Diego, California 92101, USA and Department of Radiology, University of California San Diego, San Diego, California 92093, USA
Bruce Fischl
3 CSAIL, Massachusetts Institute of Technology, Cambridge, Massachusetts
Matthew P. Frosch
4 C.S. Kubik Laboratory for Neuropathology, Massachusetts General Hospital, Boston, Massachusetts
Suzanne Corkin
5 Department of Brain and Cognitive Sciences, Massachusetts Institute of Technology, Cambridge, Massachusetts
H.M., Henry Molaison, was one of the world’s most famous amnesic patients. His amnesia was caused by an experimental brain operation, bilateral medial temporal lobe resection, carried out in 1953 to relieve intractable epilepsy. He died on December 2, 2008, and that night we conducted a wide variety of in situ MRI scans in a 3 T scanner at the Massachusetts General Hospital (Mass General) Athinoula A. Martinos Center for Biomedical Imaging. For the in situ experiments, we acquired a full set of standard clinical scans, 1 mm isotropic anatomical scans, and multiple averages of 440 µm isotropic anatomical scans. The next morning, H.M.’s body was transported to the Mass General Morgue for autopsy. The photographs taken at that time provided the first documentation of H.M.’s lesions in his physical brain. After tissue fixation, we obtained ex vivo structural data at ultra-high resolution using 3 T and 7 T magnets. For the ex vivo acquisitions, the highest resolution images were 210 µm isotropic. Based on the MRI data, the anatomical areas removed during H.M.’s experimental operation were the medial temporopolar cortex, piriform cortex, virtually all of the entorhinal cortex, most of the perirhinal cortex and subiculum, the amygdala (except parts of the dorsal-most nuclei—central and medial), anterior half of the hippocampus, and the dentate gyrus (posterior head and body). The posterior parahippocampal gyrus and medial temporal stem were partially damaged. Spared medial temporal lobe tissue included the dorsal-most amygdala, the hippocampal-amygdalotransition- area, ~2 cm of the tail of the hippocampus, a small part of perirhinal cortex, a small portion of medial hippocampal tissue, and ~2 cm of posterior parahippocampal gyrus. H.M.’s impact on the field of memory has been remarkable, and his contributions to neuroscience continue with a unique dataset that includes in vivo, in situ, and ex vivo high-resolution MRI.
INTRODUCTION
This review chronicles H.M.’s history, his contributions to the neuroscience of memory, neuroimaging studies past and present, and his autopsy. In the following paragraphs, we walk through the anatomical details of the medial temporal lobe, describe the specific structures removed and spared in H.M., and provide the only glimpse of his intact, fresh brain. We recount the critical discoveries that made him one of the most famous amnesic patients in the world, and illustrate, with high-resolution imaging, the age-related white matter disease that likely accounts for his dementia in the final part of his life. We also identify key questions to be addressed in the forthcoming neuropathological examination and in future histological studies.
On August 25, 1953, the neurosurgeon William Beecher Scoville performed an experimental operation in a 27-year-old man, Henry Gustave Molaison (H.M.), in the hope of curing his medically intractable epilepsy ( Scoville, 1954 ; Scoville and Milner, 1957 ). H.M. had experienced petit mal seizures from the age of 10 and grand mal seizures that began on his 15th birthday. The etiology of his seizures was unclear—as a young boy, he had sustained a minor head injury and, in addition, several of his father’s relatives had epilepsy. H.M. graduated from high school when he was 21 and later repaired electric motors and worked on a typewriter assembly line. He took large doses of anti-epilepsy drugs, but they did not quell his attacks. Because numerous EEG studies failed to reveal a precise surgical target for seizure control, Scoville proposed a psychosurgical procedure that he had devised, bilateral medial temporal lobotomy ( Scoville and Milner, 1957 ). He had previously performed the operation in patients with psychiatric disorders, mainly schizophrenia, with mixed results. H.M. was the first patient to undergo this procedure for intractable epilepsy. Scoville later renamed the operation bilateral medial temporal lobe resection.
Postoperatively, H.M.’s petit and grand mal attacks continued, and although their frequency decreased markedly, he required anti-epilepsy drugs for the rest of his life. His seizure control, however, was accompanied by a devastating loss. For the next 55 years, H.M. was trapped in the moment because of profound anterograde amnesia. His amnesia was pure—unconfounded by other cognitive deficits. His IQ was above average, and his language, reasoning, and perceptual capacities were normal. The exceptions were impaired olfactory function, caused by the operation, and cerebellar symptoms, a side effect of his anti-seizure medication, Dilantin.
The discrete nature and severity of H.M.’s amnesia made him the topic of scientific scrutiny for the remainder of his life and even after his death. Over 100 researchers participated in collaborative projects to study him, integrating behavioral testing, standardized interviews, and structural and functional imaging. In 1955, Brenda Milner conducted the first postoperative psychological testing of H.M., providing quantitative evidence of profound memory loss with preserved intelligence and immediate memory ( Scoville and Milner, 1957 ). She and Scoville concluded, “The findings reported herein have led us to attribute a special importance to the anterior hippocampus and hippocampal gyrus in the retention of new experience” (p. 21). Milner later introduced the idea that some memory processes were not hippocampus dependent by showing that H.M.’s error scores decreased across three days of testing on a motor skill-learning task, mirror tracing ( Milner, 1962 ). This discovery constituted the first experimental demonstration of preserved learning in amnesia.
Dissociable Memory Processes
Subsequent research with H.M. extended Milner’s pioneering work and established several firm conclusions. The evidence supported the dual process theory of memory proposed by James (1890) and Hebb (1949) . They viewed short-term and long-term memory as separate processes. Accordingly, H.M.’s short-term memory was preserved, while his long-term memory was impaired. His episodic and semantic learning were both deficient, indicating overlapping neural substrates ( Gabrieli et al., 1988 ; Steinvorth et al., 2005 ). Tests that distinguished two forms of recognition memory—recollection and familiarity—revealed that H.M. could make familiarity-based judgments to recognize complex pictures, even six months after encoding. This surprising result showed that recollection depends on the hippocampus, but familiarity does not. Examinations of H.M.’s retrograde amnesia led to the discovery that he could remember only two preoperatively experienced autobiographical episodes, whereas his semantic memory for the same time period was normal ( Steinvorth et al., 2005 ; Corkin, 2013 ). This dissociation implicates the hippocampus as necessary for the retrieval of premorbid autobiographical but not semantic information. At the same time, certain kinds of nondeclarative learning—motor skill learning, classical conditioning, and repetition priming—were preserved.
The issues that motivated decades of research with H.M. were to understand the scope of his amnesia, to elucidate the kinds of learning and memory that were spared, and to establish a causal link between his amnesia and specific brain circuits. Some information about the integrity of his brain was available even before his operation. H.M. had a pneumoencephalogram in 1946 and another in 1953, both of which were read as normal. At the time of his operation in 1953, information about the damage to his brain came exclusively from Scoville’s account of what he had removed. His notes and drawings formed the basis of a set of detailed drawings by another neurosurgeon, Lamar Roberts, which accompanied Scoville and Milner’s 1957 paper ( Scoville and Milner, 1957 ). Scoville estimated that the medial temporal lobe resection extended 8 cm back from the tip of each temporal lobe, but subsequent MRI scans indicated that the removal was much less extensive.
Postoperative In Vivo Imaging
CT scans carried out in 1977 and 1984 showed metallic clips from the operation, minimal atrophic change in the anterior temporal region bilaterally, cerebellar atrophy, and, in the 1984 scan when he was 58, mild to moderate cortical atrophy ( Corkin, 1984 ). Specific brain structures were not visualized. A SPECT scan conducted in 1992 at Brigham and Women’s Hospital in Boston confirmed his bilateral medial temporal lobe resection and cerebellar atrophy.
H.M.’s first MRI scans occurred in 1992 at Brigham and Women’s Hospital and in 1993 at Mass General, when he was 66 and 67 years old, respectively ( Corkin et al., 1997 ). These images showed that the removal extended back about 5.4 cm from the tip of the temporal lobe on the left and about 5.1 cm on the right. The bilaterally symmetrical lesion damaged most of the amygdaloid complex, the entorhinal cortex, part of perirhinal cortex, the uncal and rostral portions of the hippocampal complex, and part of parahippocampal cortex. Some of the ventral perirhinal and posterior parahippocampal cortices were intact. Approximately 2 cm of caudal hippocampal tissue was also spared, but it appeared atrophic and was likely deafferented due to removal of the entorhinal cortex. The subcortical white matter associated with the most anterior portions of the superior, middle, and inferior temporal gyri may have been compromised by the resection. The cerebellar atrophy was dramatic, but the cortical surface appeared normal for H.M.’s age ( Corkin et al., 1997 ).
A decade later, in 2002 to 2004, Salat and colleagues scanned H.M. at the Mass General Martinos Center, using improved MRI data acquisition and analysis tools—higher resolution, quantitative measures of tissue morphometry, and indices of tissue integrity ( Salat et al., 2004 , 2006 ; van der Kouwe et al., 2005 , 2006 ; Wiggins et al., 2006 ). By then, H.M. was 74 to 77 years old, and we uncovered new age-related abnormalities that were not connected to his 1953 resection—cortical thinning and abnormal signal in white matter and deep gray matter. H.M.’s T1 morphometry images showed significant atrophy of the cerebral ribbon, ranging from ~0.3 mm to ~0.7 mm relative to control participants. The atrophy that occurred between 1998 and 2003 was greater than that between 1993 and 1998, suggesting an aging-related degenerative process ( Salat et al., 2006 ). T1-weighted images also revealed infarcts in a number of subcortical gray matter structures, including the thalamus and putamen. Consistent with earlier imaging studies, H.M.’s cerebellum was severely atrophied. In T2-weighted images, Salat et al. noted significant white matter hyperintensities throughout H.M.’s brain that were especially pronounced in the inferior frontal gyrus near the corpus callosum. These new abnormalities appeared to be the result of high blood pressure and small vessel disease. We also collected the first diffusion MRI scans of H.M.’s brain, allowing the examination of fractional anisotropy (FA) maps to quantify the microstructural integrity of the white matter. Overall, H.M. had decreased FA compared to matched controls, and the focal areas of white matter damage had reduced FA. We never found any abnormality that would account for his original seizure disorder.
Current Study
H.M. died on December 2, 2008. That night, we conducted a wide variety of in situ MRI scans in a 3 T scanner at the Mass General Athinoula A. Martinos Center for Biomedical Imaging. The next morning, H.M.’s body was transported to the Mass General Morgue where Matthew Frosch, Director of the Neuropathology Unit, performed an autopsy. Jacopo Annese assisted with the autopsy. Photographs taken immediately afterward provided the first documentation of H.M.’s lesions in his physical brain. After ~10 weeks of tissue fixation, we obtained ex vivo structural data at ultra-high resolution using 3 T and 7 T magnets. This postmortem research had two goals—to document the specific structures that were removed and spared in H.M.’s brain, based on the gross examination of the fresh brain and analysis of the MRI images, and to relate the behavioral dissociations documented during H.M.’s life to the precisely established sparing and loss of brain tissue. An additional motivation for the ex vivo imaging was to provide an MRI-based method to later register the histological sections in 3D. The Partners Human Research Committee approved all studies described here. In this report, we first introduce the anatomical structures that define the medial temporal lobe region and then describe H.M.’s autopsy and MRI results.
MATERIALS AND METHODS
Participant, h.m.
At the time of his death, H.M. was 82 years old. The cause of death was arteriosclerotic cardiovascular disease. In 1992, H.M. and his court-appointed conservator had signed a brain donation form authorizing Mass General and MIT to perform a postmortem examination upon his death, and the conservator gave consent for the autopsy the evening H.M. died.
Neuroanatomy of the Intact Adult Medial Temporal Lobe—Terminology
To establish the terminology used in this report, we first describe pertinent structures in an intact adult brain, focusing on the medial temporal lobe region ( Rosene and Van Hoesen, 1987 ; Gloor, 1995 ; Insausti et al., 1995 ; Van Hoesen, 1995 ; Insausti et al., 1998 ). To educate the reader on the relevant structures, we selected nine blockface images from a control case (60 year old, male) in our MGH brain collection and labeled them ( Fig. 1 ). The areas include the piriform cortex (primary olfactory cortex), mesocortices of the parahippocampal gyrus (entorhinal and perirhinal cortices), and temporal polar cortex; the hippocampal formation—hippocampus, subiculum, and dentate gyrus; and the subcortical collection of nuclei that comprise the amygdala ( Fig. 1 ). The structures and slices are described from anterior to posterior.

Blockface images of medial temporal lobe structures from a control case (60-year-old, male). Nine levels represent the anterior-posterior extent of the medial temporal lobe. Level (A) temporal pole, (B) pyriform (olfactory) cortex, anterior-most entorhinal cortex, (C) anterior amygdala, (D) mid-amygdala, (E) anterior hippocampal head (pes), (F) posterior hippocampal head, (G) posterior hippocampal head and anterior hippocampal body, (H) hippocampal body, (I) posterior hippocampal body, hippocampal tail (not illustrated). Numerical labels correspond to Brodmann areas, and letter abbreviations are defined as: AAA = anterior amygdala area, AB = accessory basal nucleus of amygdala, B = basal nucleus of amygdala, CA = cornu ammonis (1–4), CAT = cortical amygdala transition area, Ce = central nucleus of amygdala, Co = cortical nucleus of amygdala, CS = collateral sulcus, DG = dentate gyrus, ES = endorhinal sulcus, fm = fimbria, HATA = hippocampal amygdala transition area, HF = hippocampal fissure, L = lateral nucleus of amygdala, LV = lateral ventricle, M = medial nucleus of amygdala, Pre = presubiculum, Par = parasubiculum, SUB = subiculum, TP = temporal pole, and Un = uncus. Magnification bar = 1 cm.
The temporal pole is divided into four regions: dorsal, ventral, medial, and lateral. Anteriorly, the temporal polar cortex hangs unattached to other brain tissue for ~1.5 cm ( Fig. 1A ), after which the temporal lobe joins the frontal cortex at the level of the limen insula. At this level, several features are noteworthy. The frontal insula and temporal insula merge together, the temporal polar cortex ends, and the piriform cortex begins to occupy the medial temporal area ( Fig. 1B ). The sulcal configuration in this region is complicated because the rhinal sulcus is incipient in humans and not present in all brains. The main sulci that outline the parahippocampal gyrus include the rhinal sulcus anteriorly, albeit variably, and the collateral sulcus laterally. The control case illustrated in Figure 1 does not exhibit a rhinal sulcus. If a rhinal sulcus were present, it would reside approximately at level B, at the level of the piriform cortex. Deep to the parahippocampal gyrus, the endorhinal sulcus dorsally separates the corticomedial nuclei of the amygdala from the optic tract area, the sulcus semi-annularis separates the medial parahippocampal cortex from the corticoamygdala-transition-area, and the hippocampal fissure separates the parahippocampal gyrus from the hippocampus ( Figs. 1C–F ). At the point where the frontal and temporal lobar regions connect, the temporal stem, one of the major white matter conduits for the temporal lobe, appears.
The amygdala lies posterior and slightly dorsal to the piriform cortex, which is situated deep beneath the parahippocampal cortex between the olfactory cortex anteriorly and the hippocampus posteriorly. The key amygdala nuclei are (from lateral to medial) the lateral nucleus, basal nucleus, accessory basal nucleus, paralaminar nucleus, medial nucleus, cortical nucleus, and corticoamygdala-transition-area ( Figs. 1C,D ). At the medial most edge of the amygdala, three nuclei line up from superior to inferior: medial nucleus, cortical nucleus, and corticoamygdala-transition area ( Figs. 1C,D ).
At the amygdala’s broadest part, immediately anterior and slightly dorsal to the hippocampus, the parahippocampal gyrus is also at its largest width. The anterior parahippocampal gyrus contains two Brodmann areas, area 34 medially and area 28 laterally ( Brodmann, 1909 ; Lorente de No, 1934 ). Brodmann area 34 corresponds to the gyrus ambiens and sometimes has a bulbar configuration that is often mistaken for the uncus of the hippocampus, but the uncus resides deep to the gyrus ambiens and slightly posterior ( Fig. 1D ). The hippocampal fissure borders the uncus and lower bank of the parahippocampal gyrus where the subicular cortices are located ( Fig. 1E ). Brodmann area 28 makes up a substantial component of the parahippocampal territory and occupies the entire crown of the anterior parahippocampal gyrus, commonly referred to as the entorhinal cortex. Equally prominent within the parahippocampal cortex is the entorhinal cortex’s neighbor laterally, the perirhinal cortex ( Figs. 1B–I ). The perirhinal cortex (Brodmann area 35, Braak’s transentorhinal) ( Braak and Braak, 1985 ) is sometimes slightly larger than the entorhinal cortex and surrounds it anteriorly, laterally, and posteriorly ( Insausti et al., 1998 ; Van Hoesen et al., 2000 ; Ding and Van Hoesen, 2010 ). The perirhinal cortex lies lateral to the rhinal sulcus but medial relative to the collateral sulcus, once the collateral sulcus has begun ( Van Hoesen et al., 2000 ; Ding et al., 2009 ). The ectorhinal cortex (Brodmann area 36) is temporal isocortex; we classify it separately from perirhinal cortex based on the fact that perirhinal area 35 is agranular and dysgranular (area 35a periallocortex and 35b proisocortex, respectively), whereas temporal isocortex (area 36) contains a granular layer. On the crown of the posterior parahippocampal gyrus, areas TH and TF ( von Economo and Koskinas, 1925 ; von Bonin and Bailey, 1947 ) make up the remaining parahippocampal cortex as it ends caudally at the retrosplenial cortex and calcarine sulcus (TH-TF, not illustrated).
The hippocampal formation comprises the hippocampus proper, subicular cortices (subiculum, presubiculum, and parasubiculum), and dentate gyrus ( Rosene and van Hoesen, 1987 ). The hippocampus proper contains subfields CA1, CA2, CA3, and CA4, named for cornu ammonis because it resembles a ram’s horn. The hippocampus, which contains four main structural parts, genu ( Fig. 1D ), head ( Figs. 1E–G ), body ( Figs. 1G–I ), and tail (not illustrated), sits deep beneath the parahippocampal cortex. Its structure changes significantly from anterior to posterior, with the head being disproportionately larger than the body and tail. The head of the hippocampus is made up of several convolutions, the pes hippocampi, where the medial-most convolution defines the uncus ( Figs. 1E,F ). The inferior horn of the lateral ventricle makes its first appearance at the level of the amygdala and hippocampal head.
Autopsy and Fixation
On December 4, 2008, when the postmortem interval was ~19 hrs, Matthew Frosch conducted the autopsy. H.M.’s brain was fixed in standard 10% formalin for several hours and was then transferred to buffered 4% paraformaldehyde. The fixative solution was changed twice during the two months that it remained in the Mass General Department of Pathology, allowing the brain tissue to fix thoroughly. On February 12, 2009, when it was transferred to the Martinos Center for ex vivo scanning, it remained in 4% paraformaldehyde.
In Situ MRI Acquisition
On the evening of December 2, 2008, just under 4 hr after H.M.’s death in Windsor Locks, Connecticut, we collected in situ scans at the Mass General Martinos Center. In situ refers here to postmortem imaging of the brain in the head. Images were collected in a 3 T Siemens (Erlangen, Germany) TIM Trio MRI scanner with a 32-channel head coil. We determined beforehand that the configuration of this system (in particular, the imaging gradient switching and RF energy deposition) would not damage the brain by heating the tissue or by heating or vibrating the surgical clips.
Because subject fatigue and motion were not an issue and scanning could continue for several hours, we collected high-quality, high-resolution images that would not be possible in a living subject. The in situ 3 T session lasted 9 hr. We obtained a wide range of contrasts of the unfixed brain with different scan types and high-resolution anatomical images.
We first acquired a full set of standard clinical scans for comparison with antemortem images. In addition, we obtained a 2 mm isotropic diffusion scan, 1 mm isotropic single and multiecho MPRAGEs ( Mugler and Brookeman, 1990 ; van der Kouwe et al., 2008 ), 1 mm isotropic multi-flip angle multiecho FLASH scans with 2 mm isotropic B1± maps, and multiple averages of a 440 µm isotropic single-echo multi-flip angle FLASH scan. All scans were automatically localized for acquisition using AutoAlign ( van der Kouwe et al., 2005 ; Benner et al., 2006 ). The synthetic images presented in this report were generated using estimates of intrinsic tissue parameters derived from a combination of acquired multiecho FLASH images with native contrast ( Fischl et al., 2004 ).
Clinical scans
We obtained five standard clinical scans: (1) sagittal T1-weighted spin-echo, (2) axial T2-weighted turbo-spin-echo, (3) axial FLAIR, (4) susceptibility weighted imaging (SWI), and (5) diffusion with matching B0 field map. Prescan intensity normalization was applied to all scans.
- T1-weighted 2D-encoded spin-echo ( T acq 4 m 20 s); 24 sagittal slices of 5 mm with 1 mm gap, 240 mm field of view with 256 matrix and 75% phase resolution, phase encode AP (1.3 × 0.9 × 5 mm). TE 7.1 ms, TR 550 ms, BW 201 Hz/px, FA 120°, two concatenations.
- T2-weighted 2D-encoded turbo-spin-echo ( T acq 5 m 27 s); 23 axial slices of 5 mm with 1 mm gap, 230 × 172.5 mm field of view with 512 matrix and 75% phase resolution, phase encode RL (0.6 × 0.4 × 5 mm). TE 91 ms, TR 5 s, BW 100 Hz/px, FA 134°, two averages, flow compensation in slice direction.
- FLAIR 2D-encoded turbo-spin-echo ( T acq 6 m 52 s); 23 axial slices of 5 mm with 1 mm gap, 230 × 172.5 mm field of view with 384 matrix and 75% phase resolution, phase encode RL (0.8 × 0.6 × 5 mm). TE 71 ms, TR 10 s, TI 2,500 ms, BW 130 Hz/px, FA 150°, two averages, flow compensation in slice direction.
- Susceptibility-weighted 3D-encoded gradient echo ( T acq 6 m 59 s). 96 axial partitions (k-space slices) of 1.5 mm, 220 × 171.9 mm field of view with 448 × 350 matrix, phase encode RL (0.5 × 0.5 × 1.5 mm). TE 20 ms, TR 28 ms, BW 120 Hz/px, FA 15° (slab-selective), flow compensation.
Standard anatomical scans for morphometry
We collected a standard set of 1 mm isotropic anatomical scans designed to elucidate brain morphometry using FreeSurfer ( http://surfer.nmr.mgh.harvard.edu ) ( Dale et al., 1999 ; Fischl et al., 2002 , 2004 ). The set consisted of T1-weighted multiecho MPRAGE (MEMPR) for cortical modeling and subcortical segmentation, multiecho FLASH (MEF) for tissue parameter quantification, and T2-SPACE and FLAIR T2-SPACE for T2 contrast. Prescan normalization was applied to all scans, and, for accurate alignment, scans were matched with respect to geometry and bandwidth (thus, degree of distortion).
- T1-weighted 3D-encoded 4-echo MEMPR ( van der Kouwe et al., 2008 ) ( T acq 6 m 3 s); 176 sagittal partitions of 1 mm, 256 mm field of view with 256 × 256 matrix, phase encode AP (1 mm isotropic), 2× GRAPPA with 32 reference lines. TE 1.64/3.5/5.36/7.22 ms, TR 2,530 ms, TI 1,200 ms (non-selective), BW 651 Hz/px, FA 7° (nonselective).
- T1-weighted 3D-encoded 8-echo FLASH ( T acq 13 m 25 s at each of three flip angles); 176 sagittal partitions of 1 mm, 256 mm field of view with 256 × 256 matrix, phase encode AP, 50% phase oversampling (1 mm isotropic), 2× GRAPPA with 32 reference lines. TE (1.85 + n × 2.0 + n × ( n −1)/2×0.1) ms ( n = 0,…,7) (uneven spacing for phase unwrapping), TR 22 ms, BW 651 Hz/px, FA 5/20/30° (nonselective), magnitude and phase images.
- T2-weighted variable flip angle 3D-encoded turbo-spin-echo (T2-SPACE) ( Mugler et al., 2000 ) (T acq 4 m 43 s); 176 sagittal partitions of 1 mm, 192 mm field of view with 192 × 192 matrix, phase encode AP (1 mm isotropic), 2× GRAPPA with 24 reference lines. TE 368 ms, TR 3,200 ms, BW 651 Hz/px, FA variable (nonselective).
- T2-weighted variable flip angle 3D-encoded turbo-spin-echo with fluid attenuation (FLAIR T2-SPACE) ( Mugler et al., 2000 ) ( T acq 7 m 22 s). 176 sagittal partitions of 1 mm, 192 mm field of view with 192 × 192 matrix, phase encode AP (1 mm isotropic), 2× GRAPPA with 24 reference lines. TE 352 ms, TR 5 s, TI 1,800 ms, BW 789 Hz/px, FA variable (nonselective).
T2*-weighted anatomical scans
To quantify tissue T2*, we carried out a multiecho FLASH scan according to a protocol that had a longer TR and more widely spaced echoes than the 8-echo FLASH described above. We also repeated the 8-echo FLASH protocol at 2 mm isotropic resolution (two repetitions of T acq 6 m 42 s with 128 × 128 matrix).
- 3D-encoded 8-echo FLASH ( T acq 14 m 15 s). 128 sagittal partitions of 1.33 mm, 256 mm field of view with 192 × 192 matrix, phase encode AP (1.33 mm isotropic), 2× GRAPPA with 32 reference lines. TE (3.41 + n × 6.9 + n × ( n − 1)/2 × 0.1) ms ( n = 0,․,7) (uneven spacing for phase unwrapping), TR 60 ms, BW 202 Hz/px, FA 20° (nonselective), magnitude and phase images.
High-resolution anatomical scans
We dedicated ~5 hr of the in situ scanning time to obtaining high-resolution anatomical scans, using a single-echo FLASH protocol with an isotropic resolution of 440 µm.
- T1-weighted 3D-encoded FLASH ( T acq 24 m 19 s at each of 6 flip angles); 384 sagittal partitions of 0.44 mm, 225 mm field of view with 512 × 512 matrix, phase encode AP, 50% phase oversampling (1 mm isotropic), 2× GRAPPA with 32 reference lines. TE 4.09 ms, TR 9.5 ms, BW 199 Hz/px, FA 3/5/8/10/15/18° (non-selective), magnitude and phase images.
Quality control scans
We obtained additional scans to correct artifacts. For the diffusion scans, matching B0 field maps were acquired. We collected data for 1 mm isotropic DESPOT1, DESPOT2, and HiFi analyses ( Deoni et al., 2003 , 2005 ; Deoni, 2007 ). Based on these results, we chose the optimal range of flip angles for the subsequent FLASH scans. We obtained a B1 transmit map using Actual Flip Angle Imaging ( Yarnykh, 2007 ) at 2.5 mm isotropic resolution and a B1 transmit/receive map by imaging at 2 mm isotropic resolution with the body coil and with each element of the 32-channel array. In addition, we acquired a gradient echo EPI series of volumes (TR 3 s, 192 measurements, 3 mm resolution with 0.6 mm gap between slices, 42 slices). Two additional runs used an experimental radial and Cartesian-encoded gradient echo protocol with an ultrashort echo (FLUSTER) ( Van der Kouwe, 2008 ) (data not shown).
Ex Vivo Imaging
Ex vivo imaging occurred after H.M.’s brain had been stored in 10% formalin for ~10 weeks. The fixed brain was placed in a custom-made Plexiglas chamber and imaged at high field ( Annese et al., 2014 ). We repeated the in situ scans ex vivo, and as expected the images confirmed the observations made in in situ.
High-resolution scans (7 T)
We used a 7 T Siemens scanner based on the Avanto platform for high-resolution imaging with a 31-channel custom-built head coil. The highest resolution images were 210 µm isotropic single-echo multi-flip angle FLASH scans. We measured coil covariance for image reconstruction and obtained 1.68 mm isotropic B1 transmit/receive maps and a two-echo gradient echo field map (2 mm × 2 mm × 3 mm resolution) for image correction.
We dedicated 15.5 hr of scan time to collecting high-resolution images of the entire fixed brain. Encoding at 210 µm isotropic resolution with the 31-channel head coil required offline image reconstruction because the k-space data volumes were larger than the 32 GB of RAM available on the scanner image reconstruction computer. The k -space data were streamed to an external storage site during acquisition because the total amount of data per scan exceeded the 320 GB of disk space available on the scanner RAID. We used the coil covariance matrix to combine the signals from the 31 head coil channels to form a single image volume ( Roemer et al., 1990 ).
- T1-weighted 3D-encoded FLASH ( T acq 4 h 50 m at each of three flip angles); 704 partitions of 210 µm, 175 × 153 mm field of view with 832 × 728 matrix (210 µm isotropic). TE 15.1 ms, TR 34 ms, BW 40 Hz/px, FA 10/20/30° (nonselective).
Temperature Monitoring
From the time of H.M.’s death until his body reached the Martinos Center, his head was enclosed in a Cryopak Ice Blanket to keep his brain cool. During the in situ scanning, room temperature was maintained at ~18°C. During ex vivo scanning, we monitored temperature carefully, keeping it below 19°C on the outer surface of the chamber at 3 T and 7 T. Temperature was a concern because RF energy deposition during MR imaging can heat the sample. To ensure that temperature fluctuations due to imaging would be well below normal fluctuations in room temperature, we previously monitored a test sample (whole brain) during imaging with the highest specific absorption rate sequences. A multichannel fiber optic temperature sensor (Neoptix, Inc., Quebec, Canada) recorded the temperature in four areas—deep in the tissue, close to the inner wall of the chamber, on the outer surface of the chamber, and in the room air. As expected, even during high (100%) specific absorption rate protocols, the temperature inside the tissue never increased more than 4°C relative to the inside and outside of the container. The specific absorption rate of RF energy for the 3 T diffusion sequence was close to 100% of the clinically safe value imposed by the scanner hardware, and it was substantially lower for the other protocols. The room temperature was at the minimum thermostat setting (18°C), and an additional fan was used to dissipate heat from around the chamber. The temperatures of the outer surface of the chamber and the surrounding air were monitored with the fiber optic system. Because these temperatures never exceeded 19°C, we reasoned that the temperature of the brain tissue never went above 23°C during imaging. We believe that the temperature differentials were even smaller during in situ imaging because the imaging session was shorter, the protocols were less energy intensive, and the energy could dissipate throughout the body.
Lesions in the Fresh
Brain The unfixed brain weighed 1,100 g, ~200 g less than one would expect for a 6-foot-tall, healthy man. Photographs of the brain taken immediately after its removal from the skull showed the overall topography of the gross brain to be relatively preserved. Notably, the olfactory bulbs and tracts were intact, and we saw no explicit damage, with the obvious exceptions of the medial temporal lobe excisions and a shriveled cerebellum ( Fig. 2 ). The dashed white lines drawn laterally and posteriorly illustrate where a normal sized cerebellum would be. The blood vessels appeared mildly atherosclerotic. In the right ventral temporal lobe, we identified a black surgical clip, which we believe was intentionally left behind by Scoville to prevent bleeding ( Scoville and Milner, 1957 ). A second clip was located in the left temporal lobe.

Photograph of the whole fresh brain (inferior surface) taken at H.M.’s autopsy. In the ventral view, the white arrows on both sides of the brain indicate the lines of cut in the coronal MRI slices in Figure 5 . These slices (white arrows) correspond to the in situ MRI ( Figs. 5A–L ). Note the area of excision and additional fibrous tissue (i.e., scar tissue) bilaterally, and the residual medial most tissue (bilaterally but larger on the left) in the medial temporal area, next to cranial nerve III. Abbreviations: B = basilar artery, M = medulla, MB = mammillary bodies, OB = olfactory bulb, OC = optic chiasm, ON = optic nerve, TP = temporal pole, V = vertebral artery, and III = cranial nerve III (oculomotor nerve). White arrowheads point to posterior temporal artery on both right and left. Dashed white lines illustrate atrophy in the cerebellum. Note the black surgical clip on right temporal lobe. [Color figure can be viewed in the online issue, which is available at wileyonlinelibrary.com .]
Figure 3 magnifies the ventromedial view to reveal a close-up of the right (A) and left (B) medial temporal lobe lesions. The photographs show slightly different views, a consequence of the unfixed state. (An unfixed brain collapses or widens slightly and does not retain the classical formation until fixed.) The medial temporal lobe lesions began immediately to the left and right of the optic nerves, which had been cut during the autopsy so the brain could be pulled out of the skull, leaving the eyes intact. On both sides, the lesion extended posteriorly from the temporal polar cortex through the parahippocampal gyrus until about the level of the halfway point of the basilar artery. In a normal case, the gyrus ambiens would reside immediately and slightly anterior to the uncus. In H.M., medial tissue remained on both sides but to a greater extent on the left ( Figs. 3A,B ). We could not determine definitively whether the remaining medial tissue on the left was uncus or gyrus ambiens because the banks of the hippocampal fissure and other landmarks had been surgically removed. The histological analysis, which will be the topic of a later report, will reveal whether the cytoarchitecture of this tissue is hippocampal (allocortical) or cortical (periallocortical). Continuing posteriorly, significant scar tissue occupied the medial temporal areas bilaterally.

Close-up photographs of H.M.’s medial temporal lobe showing a ventral view of the right and left temporal regions (A and B). Tissue was splayed out (due to being unfixed) to reveal a slightly different viewpoint of the extent of the lesion. In A and B, the lesion extends from the temporal pole to the midparahippocampal gyrus. Note the absence of tissue and the abundant scar tissue bilaterally. The basilar and vertebral arteries contain several atherosclerotic plaques. [Color figure can be viewed in the online issue, which is available at wileyonlinelibrary.com .]
In Situ MRI
The several different contrasts acquired in situ provided an MEMPR (T1-weighted) image ( Fig. 4A ) with the classic MRI contrast that shows CSF as dark and white matter as white. In the T2-SPACE acquisition ( Fig. 4B ), white matter was relatively dark, gray matter was brighter, and CSF was brightest. Because the lesion void was filled with CSF, it appeared bright in the image. In the T2-SPACE acquisition with fluid attenuation (FLAIR) ( Fig. 4C ), the CSF in the region of the lesion was dark, but the remaining tissue fragments were relatively bright and clearest compared to other contrasts. The bottom panels of Figure 4 show quantitative proton density (PD) ( Fig. 4D ) and T1 estimates ( Fig. 4E ) together with a synthetic image constructed from the corresponding 440 µm volumes ( Fig. 4F ) ( Fischl et al., 2004 ). We estimated the T1 and PD volumes from a combination of multiecho FLASH scans with different flip angles. Figure 4D shows only PD contrast (arbitrary units, but proportional to spin density); Figure 4E shows estimated T1 relaxation time (sec). PD reflected only the proton (or spin) density and was a relatively flat contrast. The lesions appeared fairly bright because CSF is relatively dense in free water, while the PD of white matter is slightly less than that of gray matter. Both appeared darker than CSF in the PD image. Because higher T1 produces darker voxels in T1-weighted images, the image showing the absolute T1 value had the opposite contrast of the MEMPR (i.e., white matter is darker than gray matter and CSF in the quantitative image because white matter has a shorter T1 relaxation time). To optimize contrast and increase signal-to-noise, we created a synthetic image from the FLASH scans ( Deoni et al., 2003 ; Fischl et al., 2004 ). Figure 4F shows the synthetic FLASH image that would result if the TR had been 22 ms, flip angle had been 20°, and TE had been 0. This image was synthesized from the PD and T1 estimates by applying the steady-state FLASH model, in the reverse of the estimation procedure. We chose the TR and flip angle to achieve optimal discrimination between the structures segmented by FreeSurfer ( http://www.surfer.nmr.mgh.harvard.edu/ ), based on contrast (TE 0 implies no signal decay due to T2* relaxation). The synthetic image in Figure 4F has a T1-weighted contrast similar to the MPRAGE.

Various MRI contrasts acquired at 3.0 T in situ. (A) multiecho MPRAGE (MEMPR), (B) T2-SPACE, (C) T2-SPACE FLAIR, (D) quantitative PD, (E) quantitative T1, (F) synthetic FLASH. Note the lesion in all contrasts, with the borders especially clear in the multiecho MPRAGE and synthetic FLASH images. T1 and T2-SPACE FLAIR revealed scar tissue faintly in addition to the lesion.
Lesions in medial temporal lobe structures
Here we describe the specific medial temporal lobe areas that were explicitly removed and identify other structures that remained. The 12 coronal MR images ( Fig. 5 ) highlight the lesion extent and illustrate remaining anatomical structures, based on remaining landmarks ( Figs. 5A–L ). Levels are spaced 4 mm apart. The first image shows the temporal pole where the anterior-most portion of the lesion began ( Fig. 5A ). The medial temporopolar cortex, mainly dysgranular area 38 and area 36, were removed. The temporal polar sulcus, located dorsally, was partially destroyed.

Twelve coronal MR images showing the anterior and posterior extent of H.M.’s medial temporal lobe lesion. Images were synthesized from multi-echo FLASH scans acquired in situ and are ordered from anterior (A) to posterior (L). In all images, note the enlarged ventricles, general atrophy, and a plethora of regional white matter signal abnormalities. We identify particular structures (present or absent) in each panel. A, B, C: medial temporal pole removed; D: anterior entorhinal cortex removed (i.e. piriform cortex), E: cortical amygdala and possibly central nucleus remained; F: gyrus ambiens or uncus remained; G and H: perirhinal cortex and damaged parahippocampal cortex; I: the body of the hippocampus visible; J: first full observation of parahippocampal cortex (right side) and fimbria; K: posterior tip of the lesion (right side is past the lesion), and L: the undamaged parahippocampal cortex posterior to the lesion. Magnification bar = 1 cm.
In the second image ( Fig. 5B ), the lesion entered prime temporal polar cortex territory, where we observed the optic nerves (slightly off the midline) and the caudate nucleus. Here, the limen insula had not yet connected to the frontal and temporal lobes. The temporal polar areas, 38 and area 36, were still absent at this level. The olfactory tract, seen inferiorly to the orbitofrontal gyrus, appeared normal. The third image ( Fig. 5C ) was approximately at the level of the anterior amygdala.
The fourth image ( Fig. 5D ) fell at the level of the optic chiasm. Presumptive medial structures were the posterior piriform cortex, part of perirhinal cortex (area 35), and temporal isocortical area 36. In all slices described thus far, the medial temporal stem was partially damaged bilaterally, and the white matter quality appeared compromised, with the lesion in the left hemisphere extending farther medially than in the right. The collateral sulcus appeared in images four through eight ( Figs. 5D–H ), and perirhinal cortex occupied its medial bank.
A small part of the entorhinal cortex appeared in the fifth and sixth images ( Figs. 5E,F ), but only on the extreme lateral convexity of the gyrus. The border between the entorhinal and perirhinal cortices typically falls on the corner of the parahippocampal gyrus near the collateral sulcus. The fifth image captured the emerging optic tracts, anterior commissure (midline), and caudate/putamen ( Fig. 5E ). The anterior-most amygdala—specifically parts of the endopiriform nucleus and the corticoamygdalo-transition-area—might have been present in this image. Noticeably, the anterior-amygdala-area was lacking at this level. The landmarks in the sixth image included the optic tract, anterior commissure laterally (very subtle), caudate/putamen/anterior limb of the internal capsule, globus pallidus, hypothalamus, and columns of the fornix, indicating that this slice was at the level of the mid-amygdala ( Fig. 5F ). In a healthy brain, this level would represent the amygdala at its largest extent along with the gyrus ambiens (Brodmann area 34 in humans). The left side may have contained a portion of the semilunar gyrus slightly dorsal to gyrus ambiens; this possibility will be examined in the histological analysis. Figure 5F shows the best MRI example of the medial tissue that remained. The hypothalamus appeared atrophied, likely due to the lack of hippocampal input, and the fornix and hippocampal commissure showed significant atrophy.
The seventh image is where one would expect to see the posterior amygdala, subiculum, and anterior-most hippocampus, given that other landmarks usually present at this level are visible—the mammillary bodies of the hypothalamus, the thalamus, putamen, globus pallidus, and the optic tract (tucked in medially) ( Fig. 5G ). The spared posterior hippocampal tissue first appeared in this slice, with the right side showing more hippocampal head than the left ( Fig. 5G ).
The eighth image ( Fig. 5H ) embodied the presumptive uncal hippocampus and anterior dentate gyrus level, where neighboring anatomical landmarks were the cerebral peduncles, red nucleus, substantia nigra in the brainstem, anterior nucleus of the thalamus and posterior putamen. In the ninth image ( Fig. 5I ), the right hippocampus began to resemble the classic hippocampal shape, and this slice showed the body of the hippocampus for the first time. The tenth image exposed the body of the hippocampus where the lateral geniculate nucleus of the thalamus made its first appearance, defining the end of the entorhinal cortex ( Fig. 5J ). Images ten and eleven revealed badly damaged parahippocampal gyri, especially on the left side ( Figs. 5J,K ). Images eleven and twelve showed the shrunken posterior body and tail of the hippocampus ( Figs. 5K,L ). In the twelfth image, the posterior thalamus (pulvinar), fimbria-fornix, and posterior commissure all came into view ( Fig. 5L ).
In summary, the high-resolution, high-contrast images reported here indicated that the areas removed bilaterally by suction during H.M.’s experimental operation were the medial temporopolar cortex, piriform cortex, virtually all of the entorhinal cortex, most of the perirhinal cortex (area 35), a large amount of subiculum, amygdala, except the dorsal most nuclei (i.e., central and medial), most of the hippocampus (head and body), and the dentate gyrus (posterior head and body). Parts of posterior parahippocampal gyrus (roughly equivalent to TH and TF) remained but were damaged. Other medial temporal lobe areas spared bilaterally were: the dorsal-most amygdala, part of the hippocampal-amygdalo-transition-area, a portion of perirhinal area 35, a medial portion of the hippocampus or gyrus ambiens, and the posterior body and tail of the hippocampus. The major sulci in the medial temporal lobe were remarkably well preserved bilaterally, with the exception of the hippocampal fissure. The collateral sulci were partially present, but shallow. The endorhinal sulci were present dorsally and medially, the sulci semi-annularis were spared medially, but the rhinal sulci were absent rostrally.
Ex Vivo MRI
At higher field strength (7.0 T), we acquired ultra-high-resolution images at 210 µm for the whole brain. Because the brain was suspended in 4% paraformaldehyde during scanning, the background contained some MRI signal from the water molecules in the solution. The contrast to noise ratio (CNR) and signal to noise ratio (SNR) were compromised for two reasons—the tissue contrast and solution contrast were similar, and the resolution was high. Due to the preciousness of this brain, we chose not to subject it to a proton-free liquid, such as Fomblin. Had we used a proton-free liquid, we would have avoided the unsatisfactory background observed in the 7.0 T images ( Fig. 6 ). Even with the high background, however, the ex vivo scans showed new details, and we delineated specific landmarks, such as the endorhinal sulcus and collateral sulcus, which helped determine the exact boundaries of the lesion. We outlined the lesion with dotted lines at three pertinent anterior-posterior levels: the anterior commissure, columns of fornix, and mammillary bodies. The high-resolution ex vivo scans provided additional information about the exact shape of H.M.’s lesions.

Ex vivo images of medial temporal lobe areas acquired at 7 T. Images at 210 µm isotropic show the extent of the lesion and remaining medial structures. For level-of-cut cross reference, see Figure 5 . Panels A, B, and C correspond to the MRI slices in Figures 5E–G . A shows H.M.’s lesion approximately at the level of the amygdala. The lesion shape is relatively uniform at this level. Note the square corners at the dorsal-most part of the lesion. B demonstrates the lesion where the posterior amygdala-anterior hippocampal level would have been. Note the fornix columns medially in the hypothalamus and the irregular lesion shape. The large round black structures in C are regions of susceptibility surrounding air bubbles. C illustrates the lesion at the level of the mammillary bodies where the head of the hippocampus (i.e., pes hippocampus) would normally reside. At level C the lesion narrows. The shape of the lesion changes considerably from anterior to posterior, scar tissue is visible. The white arrowheads point to the collateral sulcus. Numbers represent Brodmann areas. Abbreviations: AC = anterior commissure, ES = endorhinal sulcus, FX = fornix, MB = mammillary bodies, OC = optic chiasm, RN = red nucleus, and SN = substantia nigra.
At the level of the anterior commissure, the anterior-most levels of the entorhinal cortex (i.e., the piriform cortex) and the extreme anterior parts of amygdala were removed ( Fig. 6A ). It is noteworthy that at this level, the lesion represented a rectangular shape and showed sharp 90° angles at the innermost (and superior) borders.
The second level is at the columns of the fornix ( Fig. 6B ). At this mid-amygdala level, the lesion shape, indicated by the dotted white lines, became irregular, especially on the left ( Fig. 6B ). The white arrow points to tissue that was likely the anterior hippocampus.
The third medial temporal lobe level is at the mammillary bodies of the hypothalamus, where the hippocampal head is largest. The right lesion appeared significantly larger than the left in the medial-lateral direction, consistent with our lesion measurements. Based on the quantitative data and these qualitative data, the right-sided lesion was slightly shorter than the left-sided in the anterior-posterior plane but slightly wider in the medial-lateral plane.
Lesion Size
We defined the overall length and width of H.M.’s lesion as the distance parallel to the anterior-posterior direction and left-right direction, respectively. We considered the lesion measurements in two ways, the total ablation and the total ablation plus damaged cortex. For the left-sided lesion, the length from the tip of the temporal lobe was 4.6 cm for the ablation and 6.0 cm for the ablation and damaged cortex ( Fig. 7 ). For the right-sided lesion, the length from the tip of the temporal lobe was 4.2 cm for the ablation and 5.5 cm for the ablation and damaged cortex ( Fig. 7 ). These dimensions indicated that the left medial temporal lobe lesion was larger than the right. The width was 2.04 cm on the left and 1.89 cm on the right at the temporal pole, 1.54 cm on the left and 1.63 cm on the right at the amygdala/uncus, and 1.02 cm on the left and 0.95 cm on the right at the hippocampal tail. The overall shape of the lesion matched a truncated cone with the wide base anterior and the narrow end posterior.

Axial view of the multiecho FLASH images. Line measurements were acquired in the axial plane to assess the anterior-posterior lesion size. The ablation on the right side measured 5.5 cm while the left measured 6.0 cm. The left temporal lobe lost considerably more cortex during surgery. Note the cerebellar atrophy due to Dilantin.
We also obtained two high-resolution anatomical volumes—the 440 µm in situ volume and the 210 µm ex vivo volume. Both were synthesized from multiple FLASH scans collected at different flip angles. The in situ 440 µm volume had near in vivo contrast, with gray matter appearing darker than white matter and CSF that was dark, but not black (visible also in the vicinity of the lesion).
The ex vivo 210 µm volume had a different contrast because the tissue was fixed (tissue classes are better distinguished by T2* contrast in fixed ex vivo brain tissue) ( Tovi and Ericsson, 1992 ). In these images, gray matter was brighter than white matter and exhibited distinct layers, such as the stria Gennari , which were not visible in the in situ scans. Because the brain was packed in paraformaldehyde, which generated a relatively strong signal that was comparable to white matter, the ventricles and region of the lesion were not extremely distinct from the adjacent structures. The cotton packing and the brain contained small bubbles, and the latter gave rise to spin dephasing artifacts, which were exaggerated by the choice of a long TE, a consequence of the low bandwidth needed to obtain reasonable SNR and gradient strengths at the required high resolution.
The white matter was noticeably inhomogeneous in both the in situ and ex vivo images, presumably a consequence of aging-related white matter disease. In the ex vivo images, B1 + (RF transmit field) inhomogeneities due to the dielectric resonance effect at high field contributed further to overall image non-uniformity. Despite the fact that we invested 15.5 hrs in the 210 µm volumes vs. 2.5 hrs for the 440 µm, SNR was higher for the 440 µm volume because SNR falls dramatically with increasing isotropic resolution. SNR was roughly 20 to 50 and 130 to 150 for brain tissue in the 210 µm and 440 µm volumes, respectively. These values are approximate because SNR varies spatially, and the noise distribution is not precisely Gaussian. We selected protocols that would give reasonable SNR in the time available. The CNR (gray/white) for the 440 µm data was 14.7. In the 210 µm data, CNR measures for gray/white matter were 15.1 for high signal areas and 5.7 for medium signal areas. The SNR measures in these regions were 4.24 and 3.39, respectively ( Fig. 4 ).
Structures Outside the Medial Temporal Lobes
The cerebellum was severely atrophic and at autopsy appeared nearly half the size of a healthy cerebellum. The cerebellar atrophy seemed uniform with the flocculonodulus, vermis, and lateral hemispheres all reduced in size. In the in situ MRI images, we observed the dentate nucleus, the largest of the deep cerebellar nuclei, in its usual location, but we could not detect the other nuclei (fastigial, globose, emboliform), given the resolution (440 um) 3 .
Aging-related changes
At the end of his life, H.M. became demented. Multiple small strokes due to untreated hypertension and white matter atrophy likely contributed to his mental deterioration. The black spots in the MRI images indicate hypertensive disease and were particularly notable in the brainstem in Figure 4A . As a consequence of the untreated hypertension and perhaps other aging processes, we observed extensive isocortical and subcortical atrophy in all structures. Limbic structures such as the fornix, hippocampal commissure, and mammillary bodies, which were connected to the hippocampus before the surgery, were markedly degenerated ( Fig. 5G ). Similarly, the extended amygdala (centromedial amygdala and bed nucleus of stria terminalis) and other structures (i.e., the substantia inominata, including the nucleus basalis of Meynert and the diagonal band of Broca) showed noteworthy deterioration, as did the ventral striatopallidal areas. In the basal forebrain, the atrophy was so extensive that it was difficult to discern the location of nuclei. Degeneration went beyond the limbic cortices and limbic-related areas. We observed severe atrophy in the striatopallidum (caudate, putamen, globus pallidus) and thalamus (anterior nucleus, ventral anterior nucleus, mediodorsal nucleus, lateral posterior, lateral dorsal nucleus, pulvinar nucleus, medial geniculate nucleus and lateral geniculate nucleus) as demonstrated by the large size of the third ventricle ( Figs. 5H–J ). We noted atrophy of the anterior commissure and all parts of the corpus callosum. The corpus callosum appeared so thin that it resembled the thickness of a healthy anterior commissure. We found isocortical thinning in all areas, including primary and secondary sensory and motor cortices. Shrinkage was especially pronounced in prefrontal, temporal, parietal, and occipital association areas.
White Matter Integrity
The diffusion imaging data acquired as part of the imaging sessions will be reported at a later time. The structural images, however, demonstrated a clear and substantial deterioration of the connective integrity of H.M.’s brain, with overt macrostructural white matter lesions throughout several portions of the cerebrum ( Fig. 5 ). We previously described this damage and noted that hints of white matter deterioration were apparent as far back as 1998 when he was 72 ( Corkin et al., 1997 ; Salat et al., 2006 ). In the 2008 imaging sessions, dark bands of hypointense white matter in T1 images were obvious throughout the periventricular regions. Such changes are commonly observed in older adults, but the degree of alterations here was well beyond what could be considered a benign consequence of aging.
Blood Supply
We did not explicitly trace the blood vessels in H.M.’s brain, but based on the anatomical areas that were surgically removed, it is likely that the blood supply was also interrupted. The arterial origins of the vascular supply to the medial temporal lobe include the middle cerebral artery, internal carotid artery, anterior choroidal artery, and posterior cerebral artery. From the fresh brain, it appeared that the anterior temporal artery, a branch of the posterior cerebral artery was removed bilaterally. The anterior choroidal artery, known to supply the anterior hippocampus, was absent on both sides, as was the anteroinferior parahippocampal artery, which supplies the anterior parahippocampal gyrus (i.e., entorhinal cortex). The posterior temporal artery was spared on both sides ( Figs. 2 and and3) 3 ) as well as the posterior parahippocampal artery, which supplies the posterior parahippocampal gyrus (i.e., TH and TF). Further tissue damage suggested that the posterior hippocampal artery, which supplies the posterior hippocampus, was compromised bilaterally.
The onset of H.M.’s profound memory impairment immediately after the operation established for the first time that removal of the hippocampus and surrounding structures causes amnesia ( Scoville and Milner, 1957 ). The postmortem studies reported here are part of an ongoing effort to characterize the damage as precisely as possible. Our first goal was to document in detail the medial temporal lobe lesions, and the second was to examine the integrity of other structures that likely supported his intact intellect and preserved learning capacities. Bearing this anatomical information in mind, we associate specific cognitive processes that were impaired or spared in H.M. to particular circuits inside and outside the medial temporal lobe. Many of the findings with H.M. informed ongoing controversies in cognitive neuroscience concerning dissociations of function.
This report extends previous anatomical findings in MRI by revealing more detail and specific anatomy about what structures were removed or spared. The autopsy photographs showed the dense scar tissue in H.M.’s lesion and the marked cerebellar atrophy, while the MRI data, including in situ and ex vivo images, capitalized on improved contrast and resolution to reveal the lesion shape (or remaining tissue shape) and the precise measurements for lesion size. For example, the improved contrast highlighted tissue that may be the gyrus ambiens or a sliver of the anterior uncus. It is difficult to estimate whether this tissue is gyrus ambiens (medial part of entorhinal cortex) or uncal hippocampal tissue (i.e., medial CA1) and this new finding must be evaluated histologically. The high-resolution MRI also showed contrast differences between damaged and undamaged tissue that allowed more precise measurement of overall lesion size and shape. In this report, the lesion, measured from 440 µm 3 MRI, was 6.0 cm on the right and 5.5 cm on the left, suggesting that Scoville ( Scoville and Milner, 1957 ) overestimated the lesion at 8 cm while Corkin and colleagues ( Corkin et al., 1997 ) slightly underestimated the right-sided lesion at 5.1 cm. The high-resolution MRI also revealed the shape of the lesions, which exactly followed the contour of the parahippocampal gyrus, wider anteriorly and narrower posteriorly. Previous in vivo MRI images showed that H.M. developed significant white matter damage and cortical thinning as he aged ( Salat et al., 2006 ). The new in situ MRI findings provide evidence of white matter disease progression, showing that at the time of H.M.’s death, his white matter was riddled with white matter signal abnormalities and extensive cortical thinning. Cortical thinning and white matter signal abnormalities were widespread, and no structure was spared. The following paragraphs review how H.M.’s lesions relate to the rich body of behavioral data collected over 55 years.
Lesion Dimensions
Overall lesion size.
At the time of H.M.’s operation, Scoville estimated that the lesion extended 8 cm back from the tip of the temporal lobe. If this had been the case, then the damage would have invaded visual cortex, which it did not. With the advent of MRI, we were able to get a more accurate idea of lesion size. The in vivo MRI estimates in the rostrocaudal extent, based on 1 mm MRI data, were ~5.4 cm on the left and ~5.1 cm on the right ( Corkin et al., 1997 ). The comparable postmortem MRI dimensions were slightly greater, showing that H.M.’s lesion was 6.0 cm on the left and 5.5 cm on the right ( Fig. 7 ). The high-resolution in situ data allowed us to measure the excision and the damaged tissue more precisely. In the left temporal lobe, the excised region measured 4.6 cm, the damaged area an additional ~1.5 cm, and the entire lesion 6.0 cm. On the right side, the excision measured 4.2 cm, the damaged region an additional ~1.4 cm, and the whole lesion 5.5 cm. The ex vivo measures were more accurate because of the superior resolution we could obtain in long scan sessions at 3 T and 7 T that would not have been feasible in vivo. We hypothesize that the greater lesion size was due in part to age- and disease-related degeneration. The in situ MRI findings reported here are the most accurate measures of H.M.’s lesion size because the brain had not yet been distorted by removal and fixation procedures.
Correlation between lesion size, distribution, and severity of amnesia
H.M. had both extensive amnesia and large medial temporal lesions, suggesting that amnesia severity is related to lesion size. His amnesia was more profound than that of Penfield and Milner’s patients F.C. and P.B., whose excisions for epilepsy and pre-existing damage spared a considerable amount of medial temporal tissue ( Penfield and Milner, 1958 ; Milner, 1959 ). More recent findings from Squire’s laboratory extend the evidence concerning correlations with lesion size and support the view that lesions restricted to the hippocampus produce less severe memory loss than lesions of the hippocampus plus other temporal lobe areas. R.B.’s lesion, limited to the CA1 field, resulted in moderately severe amnesia ( Zola-Morgan and Squire, 1986 ), whereas two other patients, G.P. and E.P., with damage to their entire medial temporal lobes bilaterally, were profoundly amnesic ( Stefanacci et al., 2000 ; Bayley and Squire, 2005 ). Notably, E.P.’s lesion included the temporal pole, amygdala, entorhinal cortex, hippocampus, perirhinal cortex, and rostral parahippocampal cortex and also extended into lateral temporal neocortex; his declarative memory was even more impaired than H.M.’s ( Insausti et al., 2013 ).
Medial Temporal Lobe Structures Excised and Spared
After H.M.’s death in 2008, we assessed his lesion using high-resolution in situ and ex vivo MRI. These images showed that the following areas were removed or damaged: substantial portions of the medial temporopolar, piriform, entorhinal, perirhinal, and parahippocampal cortices, as well as the subiculum, presubiculum, parasubiculum, amygdala, hippocampal fields CA1, CA2, CA3, and CA4 (in the hippocampal head and body), and dentate gyrus (posterior head and body). Further, our analyses suggested that a few noteworthy structures survived the medial temporal lobe surgery and may have been more difficult to discern with in vivo MRI scans due to lower resolution ( Corkin et al., 1997 ; Salat et al., 2006 ). Notably, several areas within the amygdala were spared—parts of the amygdalar medial nucleus, cortical nucleus, cortical amygdaloid transition area, amygdala-striatal zone, endopiriform nucleus, and a portion of the central nucleus. Also visible were the hippocampal-amygdalo-transition-area (HATA), a small portion of the uncus, the tail of the hippocampus (~2 cm), a small part of the perirhinal cortex (Brodmann area 35), the entire ectorhinal cortex (Brodmann area 36), and ~2 cm of the posterior parahippocampal gyrus. Although the residual hippocampal, perirhinal, and parahippocampal tissue was first documented in the 1992 and 1993 MRI images ( Corkin et al., 1997 ), the present findings further specify the locus and extent of the spared tissue.
Neural Substrate for H.M.’s Amnesia
Parahippocampal cortices and hippocampal formation.
After his operation in 1953, H.M. could not consolidate and retrieve new facts and events, documenting for the first time that circuits within the medial temporal lobe are necessary for the establishment of long-term declarative memory ( Scoville and Milner, 1957 ). Memory experiments carried out over the next five decades showed that H.M.’s deficits were severe and extensive, affecting the acquisition of verbal and nonverbal material presented via four sensory modalities ( Milner, 1968 ; Corkin, 1984 , 2002 ). He could not learn new episodic or semantic information ( Gabrieli et al., 1988 ; O’Kane et al., 2004 ), highlighting the critical role of medial temporal lobe structures in all kinds of declarative memory. Further support for this brain-behavior correlation came from MRI studies carried out in the 1990s, which gave a more accurate picture of the specific structures that were excised and spared in H.M.’s brain. The postmortem studies reported here provide new details of his lesions that could not be gleaned from the in vivo imaging studies.
By far the greatest territory removed on the day of surgery was the parahippocampal gyrus, in particular, the anterior parts of the perirhinal cortex and the entire entorhinal cortex. In a normal brain, the entorhinal cortex receives input from several secondary and tertiary association cortices and multimodal areas (in prefrontal cortex and superior temporal association cortex) and acts as the ultimate end station before extrinsic sensory afferents converge prior to entering the hippocampus ( Van Hoesen, 1997 ; Van Hoesen et al., 1972 ). After this convergence on the entorhinal cortex, entorhinal layer II and superficial layer III then project to the hippocampus via the perforant pathway ( Van Hoesen and Pandya, 1975 ).
Scoville’s resection included the mesocortices of the anterior parahippocampal gyrus (entorhinal and perirhinal cortices (perirhinal area 35)) and also the hippocampal head and body. As a result, H.M.’s perforant pathway was destroyed at its origin and termination, thereby eliminating the entire circuitry necessary for long-term declarative memory. The remaining ~2 cm of hippocampal tissue was deafferented, and, therefore, not able to support long-term memory formation, storage, and retrieval. A preliminary histological study showed that the remaining hippocampal tissue contained substantial gliosis, which would further compromise the residual tissue ( Annese et al., 2014 ).
H.M.’s pervasive memory impairment resulted from the removal of a significant portion of his hippocampi, including all CA subfields, the anterior dentate gyrus, anterior subiculum, anterior presubiculum, and prosubiculum. The hippocampal remnants included the hippocampal-amygdala-transition-area (HATA), the tail of the hippocampus, and a small portion of medial hippocampal tissue. Subsequent histological studies will clarify whether the small medial remnant is uncus or gyrus ambiens. Also spared were the posterior portions of the subiculum, dentate gyrus, and hippocampal body and tail. The posterior portion of the subiculum appeared to be intact, possibly leaving the subicular projections to the anterior, lateral dorsal, reuniens, and paraventricular nuclei of the thalamus untouched ( Aggleton et al., 1986 ). It is clear, however, that this modest input, if it existed, was not sufficient to support normal memory function in H.M.
A new finding in the postmortem scans concerned the medial temporal stem. In 1997, we reported that the temporal stem was intact ( Corkin et al., 1997 ), although Gaffan disagreed ( Gaffan, 2001 ). In the current study, major advances in technology allowed us to examine the temporal stem with greater precision in the in situ and ex vivo images. We noted that this structure was markedly deteriorated in H.M. in situ and showed decreased contrast in MPRAGE (see Figs. 5C–E ). It is unclear whether these lesions dated back to the surgical excision or were caused by degenerative disease late in life, but we favor the view that this damage was due to aging and white matter deterioration as noted in previous MRIs ( Salat et al., 2006 ) and not to Scoville’s original resection. The role of the temporal stem in amnesia has been somewhat controversial ( Horel, 1978 ; Gaffan et al., 2001 ; Gaffan, 2001 ), but a study by Zola-Morgan, Squire, and Mishkin ( Zola-Morgan et al., 1982 ) appeared to be irrefutable. They found that monkeys in whom the temporal stem white matter had been cut bilaterally were unimpaired on a delayed nonmatching-to-sample task, whereas animals with bilateral lesions of the amygdala, hippocampus, and parahippocampal gyrus showed severe impairment. A later study by Gaffan et al. was consistent with this view ( Gaffan et al., 2001 ). On a delayed matching-to-sample task, the performance of monkeys with transection of the anterior temporal stem alone did not differ significantly from their preoperative levels. It is, therefore, unlikely that H.M.’s temporal stem lesions contributed to his declarative memory impairment. Rather, his profound amnesia was caused by the excision of the parahippocampal cortices and hippocampal formation. H.M.’s performance on delayed-match-to-sample and delayed-nonmatch-to-sample tasks was comparable to that of control participants 6 months after learning ( Freed et al., 1987 ; Freed and Corkin, 1988 ), suggesting that his partially intact perirhinal cortex may have been engaged to carry out these tasks.
Aging, Cortical Thinning, and White Matter Damage
Previous in vivo MRIs characterized H.M.’s cortical and white matter damage ( Salat et al., 2006 ). At the time of his death, he was demented, and his entire brain was severely atrophied, with no structure escaping degeneration. As noted previously ( Annese et al., 2014 ), we observed a small lesion in the left orbitofrontal region in the in situ and ex vivo MRI scans that was not described in Scoville’s original report ( Scoville and Milner, 1957 ). The etiology of this lesion is unclear, but we are confident that planned histological studies will reveal the cause. The possibilities include damage by the retractor used to elevate H.M.’s left frontal lobe, deafferentation of a medial temporal lobe projection, or white matter disease, possibly due to small vessel ischemic disease, such as untreated hypertension. The white matter damage throughout H.M.’s brain was severe in the in situ and ex vivo images, and it was far worse than in other untreated hypertensive cases at his age (JCA and DHS, personal observation) ( Fazekas et al., 1993 ; Young et al., 2008 ). Substantial contrast changes in older adults may be due to significant dysfunction of vascular regulatory mechanisms ( Braffman et al., 1988 ; Breteler et al., 1994b ; Longstreth et al., 1996 ; Erkinjuntti, 2007 ), and given the substantial white matter abnormalities that we observed in H.M.’s brain ex vivo, it is likely that white matter damage caused his dementia.
Neural Substrates for H.M.’s Preserved Memory Capacities
H.M.’s deep and lasting amnesia attests to the fact that the spared medial temporal lobe structures were unable to support normal memory function or anything approaching it. Still, over the years, he occasionally surprised his examiners by retrieving episodic and semantic information that he encountered after his operation. The most astonishing example came from a picture recognition experiment in which we asked him to look at and remember complex colorful pictures for 20 sec each. Not only did he achieve normal recognition at 10 min, 24 hrs, 72 hrs, and 1 wk after encoding, but he also scored within 1 SD of the control mean 6 months later ( Freed et al., 1987 ; Freed and Corkin, 1988 ).
We attribute H.M.’s ability to recognize the complex pictures to the engagement of familiarity–based processes supported by his residual perirhinal and parahippocampal cortices in communication with his preserved cortical circuitry. Our MRI data obtained in vivo and in situ showed some remnants of perirhinal and parahippocampal cortices bilaterally ( Figs. 5E–G ) ( Corkin et al., 1997 ). Evidence accumulated over the last 20 years strongly suggests that recollection and familiarity engage different medial temporal lobe areas, with recollection mediated by the hippocampus and familiarity by perirhinal and parahippocampal cortices ( Aggleton and Brown, 1999 , 2005 ; Yonelinas and Jacoby, 2012 ). H.M.’s ability to recognize complex visual stimuli underscores the point that the hippocampus is not necessary for recognition memory based on familiarity.
H.M. was also able to recognize and provide a few distinguishing details about celebrities and politicians who rose to fame after his operation, such as JFK, Ray Charles, and Liza Minnelli ( Gabrieli et al., 1988 ; O’Kane et al., 2004 ). We attribute these glimmers of memory formation in part to processing in preserved medial temporal lobe structures seen in the in situ and ex vivo MRI images: part of perirhinal cortex, posterior parahippocampal cortex, dorsal-most amygdala, and the medial-most uncus. It is possible that these small pieces of medial temporal lobe that remained, especially posteriorly, helped support declarative memory formation on rare occasions. H.M. spent a lot of time watching television and leafing through magazines, which exposed him to a wealth of information about celebrities. This repeated stimulation over months and years enabled him to build up meager representations of a handful of famous people; he acquired this knowledge slowly over time and not via the fast declarative memory processes that healthy individuals would employ.
H.M.’s preserved memory capacities also included those now classified as nondeclarative. Milner’s 1962 groundbreaking report that he showed procedural learning over three days introduced the idea that the human brain houses dissociable memory circuits ( Milner, 1970 ). Subsequent experiments extended this result, showing that H.M. could acquire a variety of motor skills ( Corkin, 1968 ). Studies in patients with Parkinson disease, Huntington disease, and cerebellar degeneration later indicated that the striatum and cerebellum mediate motor skill learning ( Sanes et al., 1990 ; Knopman and Nissen, 1991 ; Pascual-Leone et al., 1993 ; Breteler et al., 1994a , b ; Corkin, 2013 ). Our in vivo MRI results confirmed that the striatum was not damaged in H.M.’s operation, and although his cerebellum was atrophied, it did not appear grossly abnormal.
Subsequent studies evaluated H.M.’s performance on other kinds of nondeclarative memory tasks. In a series of eyeblink classical conditioning experiments, he acquired conditioned responses in both the delay and trace paradigms ( Woodruff-Pak, 1993 ). Although he required more trials to reach criterion than his control, the fact that he showed any conditioned responses is a challenge to explain because previous research has established that the cerebellum, hippocampus, and amygdala play a major role in eyeblink conditioning (Woodruff-Pak et al., 1985; Weisz et al., 1992 ; Thompson and Kim, 1996 ; Clark et al., 2002 ; Christian and Thompson, 2003; Thompson and Steinmetz, 2009). In H.M.’s brain, the hippocampus and amygdala were extirpated, and the cerebellum was markedly atrophied. Still, it is possible that his cerebellum and deep cerebellar nuclei supported the learning, and we will examine these structures microscopically in hope of uncovering clues about his ability to acquire conditioned responses.
Repetition priming refers to a kind of learning in which recent incidental exposure to test stimuli, such as words, pictures, and patterns, facilitates subsequent processing of that information. Priming is evidence that past experience can influence memory unconsciously, that is, when participants are not trying intentionally to recall the past, and it is mediated by cortical pathways undamaged in H.M. In several experiments, he demonstrated intact performance on perceptual and conceptual priming tasks ( Gabrieli et al., 1990 , 1995 ; Keane et al., 1995 ). Companion studies in patients with cortical lesions indicated that conceptual priming is mediated by lateral temporal and parietal circuits, while perceptual priming depends on occipital circuits ( Keane et al., 1991 , 1994 , 1995 ; Gabrieli et al., 1994 ). These cortical networks were intact in H.M. and likely the underpinnings of his intact priming performance. This finding of preserved nondeclarative memory considered side by side with his impoverished declarative memory, measured by tests of recall and recognition, established the validity of cognitive and neural dissociations among memory processes.
Neural Substrates for H.M.’s Nonmnemonic Behavioral Deficits
Examination of H.M.’s fresh brain at autopsy indicated that his olfactory bulbs and tract were undamaged, but the surgical removal did include primary olfactory cortex in the temporal lobe (piriform cortex and periamygdaloid cortex). As a result, H.M. was anosmic. Extensive behavioral testing uncovered limited preserved function and severe deficits on several olfactory tasks ( Eichenbaum et al., 1983 ). His detection of weak odorants was normal as was his threshold for discrimination of intensity differences, and he showed normal adaptation to a strong odor. In contrast, his ability to discriminate odor quality was completely absent on three different measures: signal-detection testing, the triangle match-to-sample task, and a common-odor-matching task, likely due to the absence of piriform and periamygdaloid cortices ( Eichenbaum et al., 1983 ). This striking dissociation of olfactory perceptual capacities established that odor quality discrimination and recognition are not necessary for detection, intensity discrimination, or adaptation.
The in situ and ex vivo MRI studies described here brought to light the details of H.M.’s extensive amygdala resection, which included the lateral, basolateral, accessory basal, and paralaminar nuclei, a portion or all of the central nucleus, and the anterior amygdala area. The result was that most of H.M.’s amygdala output was silenced, and it is likely that the amygdala resection explains a cluster of behavioral abnormalities. H.M.’s perception of pain was diminished in the laboratory and in daily life, he showed no change in his ratings of hunger and thirst from before to after a meal, he was asexual, and he was not fearful of anything ( Hebben et al., 1985 ; Corkin, 2013 ). Nevertheless, he could experience and display a range of emotions, such as happiness, friendliness, sadness, worry, guilt, and aggression, and he was able to label the emotion in various facial expressions ( Corkin, 2013 ). The emotional response system is complex, and the underlying brain mechanisms connecting inputs and outputs engage cortical and subcortical circuits beyond the amygdala ( Price, 2003 ). A goal of future histological studies will be to examine the integrity of these connections and remnants of the amygdala in H.M.’s brain.
Neural Substrate for H.M.’s Preserved Cognitive Capacities
The MR images collected from H.M. in 1992 and 1993, four decades after his operation, showed that his frontal, parietal, and occipital cortices were normal for his age, as was the lateral temporal neocortex. At that time, it was unclear whether the subcortical white matter associated with the most anterior portions of the superior, middle, and inferior temporal gyri was damaged, but our in situ and ex vivo images confirmed that these tracts were abnormal. Nevertheless, the vast expanse of cortex on both sides of H.M.’s brain likely functioned near optimally, allowing the engagement of multiple specialized circuits to support his performance on a broad spectrum of cognitive tasks.
Milner conducted H.M.’s first postoperative psychological examination in 1955, 2 years after his operation ( Scoville and Milner, 1957 ). On the Wechsler-Bellevue Intelligence Scale, he achieved an IQ of 112, but on the Wechsler Memory Scale, his MQ was only 67, indicating normal intelligence coupled with markedly impaired long-term memory ( Wechsler, 1945 ). On subsequent testing with different forms of the Intelligence Scale and Memory Scale, he maintained this pattern of performance through 2000 ( Kensinger et al., 2001 ). This longitudinal analysis firmly established that medial temporal lobe structures are not necessary for optimal performance on IQ tests, indicating a clear dissociation between high-order cognition and long-term declarative memory.
The high-resolution, high-contrast MRI methods highlighted here confirmed that H.M.’s cortical and subcortical language areas remained intact following his surgery, and the results from numerous experimental measures and standardized tests showed that his language functions were largely spared ( Kensinger et al., 2001 ). He successfully completed seven lexical memory tasks: spelling; picture naming, name recognition, and information retrieval; Boston naming test; picture naming; picture judgment; category identification; and landmark identification, and on tests of morphology, he was able to produce and judge regular and irregular inflectional or derivational forms, including plural production, past-tense production, past tense judgment, and derivational morphology production. Two additional tasks measured his syntax processing, and he performed them normally. In general, H.M. maintained his preoperative lexical knowledge without explicit retraining, indicating that medial temporal lobe structures are not necessary for the retention of already learned lexical information. They are, however, critical for the acquisition of new lexical information (e.g., new vocabulary, celebrities) ( Gabrieli et al., 1988 ; Postle and Corkin, 1998 ). Notable exceptions in the language domain were H.M.’s impaired performance on fluency tasks and uneven success in detecting linguistic ambiguities ( Lackner, 1974 ; Corkin, 2013 ). These deficits likely stemmed from a combination of factors: minimal surgical damage to anterolateral temporal cortex, slow responding, substandard education, and lower socioeconomic background ( Kensinger et al., 2001 ; Schmolck et al., 2002 ).
Evidence of preserved problem solving and working memory processes came from H.M.’s consistently excellent performance on the Wisconsin Card Sorting Test ( Milner, 1968 ). During each administration of the task over years of testing, he quickly changed to a new sorting category as needed and had very few perseverative errors, but he was always unaware that he had done the test before. To perform this complex task, H.M. had to recruit multiple cognitive processes and engage circuits in prefrontal cortex and posterior parietal cortex, areas that were spared in the 1953 surgery ( Corkin et al., 1997 ; Salat et al., 2006 ).
H.M.’s bilateral medial temporal lobe resection was circumscribed, and the resulting amnesia was pure. He revolutionized the science of memory through his participation in numerous behavioral and imaging studies, and he continues to illuminate the science of memory. During his lifetime, neuroimaging advanced with specialized sequences and sophisticated multichannel array coils that enabled high resolution MRI. These tools allowed a final and riveting inspection of H.M.’s lesions and remaining anatomy. This postmortem research is consistent with his wishes: He knew he was contributing to science and gladly donated his brain for future study. His mantra was, “Whatever is beneficial.” It is fitting that the field of neuroscience continues to benefit from his contributions, even after his death.
Acknowledgments
The authors are grateful to H.M. and his conservator for the generous tissue donation. The authors also thank M. Dylan Tisdall, Jonathan R. Polimeni, and Thomas Witzel for technical assistance in developing protocols to accommodate extremely large data files and Kristen Huber for photographing the blockface images and laboratory preparations.
Grant sponsor: National Center for Research Resources; Grant number: P41-RR14075; Grant sponsor: NCRR BIRN Morphometric Project; Grant numbers: BIRN002 and U24 RR021382; Grant sponsor: National Institute for Biomedical Imaging and Bioengineering; Grant number: R01EB006758; Grant sponsor: National Institute on Aging; Grant numbers: AG022381 and 5R01AG008122-22; Grant sponsor: NIH Blueprint for Neuroscience Research; Grant number: 5U01-MH093765; Grant sponsors: Part of the Multi-Institutional Human Connectome Project and National Science Foundation; Grant number: NSF-SGER0714660; Grant sponsor: Dana Foundation; Grant number: 2007-4234.
- Aggleton JP, Brown MW. Episodic memory, amnesia, and the hippocampal-anterior thalamic axis. Behav Brain Sci. 1999; 22 :425–444. discussion 444–489. [ PubMed ] [ Google Scholar ]
- Aggleton JP, Brown MW. Contrasting hippocampal and perirhinal cortex function using immediate early gene imaging. Q J Exp Psychol B. 2005; 58 :218–233. [ PubMed ] [ Google Scholar ]
- Aggleton JP, Desimone R, Mishkin M. The origin, course, and termination of the hippocampothalamic projections in the macaque. J Comp Neurol. 1986; 243 :409–421. [ PubMed ] [ Google Scholar ]
- Annese J, Schenker-Ahmed NM, Bartsch H, Maechler P, Sheh C, Thomas N, Kayano J, Ghatan A, Bresler N, Frosch MP, Klaming R, Corkin S. Postmortem examination of patient H.M.’s brain based on histological sectioning and digital 3D reconstruction. Nat Commun. 2014; 5 :3122. [ PMC free article ] [ PubMed ] [ Google Scholar ]
- Bayley PJ, Squire LR. Failure to acquire new semantic knowledge in patients with large medial temporal lobe lesions. Hippocampus. 2005; 15 :273–280. [ PMC free article ] [ PubMed ] [ Google Scholar ]
- Benner T, Wisco JJ, van der Kouwe AJ, Fischl B, Vangel MG, Hochberg FH, Sorensen AG. Comparison of manual and automatic section positioning of brain MR images. Radiology. 2006; 239 :246–254. [ PubMed ] [ Google Scholar ]
- Braak H, Braak E. On areas of transition between entorhinal allocortex and temporal isocortex in the human brain. Normal morphology and lamina-specific pathology in Alzheimer’s disease. Acta Neuropathol. 1985; 68 :325–332. [ PubMed ] [ Google Scholar ]
- Braffman BH, Zimmerman RA, Trojanowski JQ, Gonatas NK, Hickey WF, Schlaepfer WW. Brain MR: Pathologic correlation with gross and histopathology. 2. Hyperintense white-matter foci in the elderly. AJR Am J Roentgenol. 1988; 151 :559–566. [ PubMed ] [ Google Scholar ]
- Breteler MM, van Amerongen NM, van Swieten JC, Claus JJ, Grobbee DE, van Gijn J, Hofman A, van Harskamp F. Cognitive correlates of ventricular enlargement and cerebral white matter lesions on magnetic resonance imaging. The Rotterdam Study. Stroke. 1994a; 25 :1109–1115. [ PubMed ] [ Google Scholar ]
- Breteler MM, van Swieten JC, Bots ML, Grobbee DE, Claus JJ, van den Hout JH, van Harskamp F, Tanghe HL, de Jong PT, van Gijn J, et al. Cerebral white matter lesions, vascular risk factors, and cognitive function in a population-based study: The Rotterdam Study. Neurology. 1994b; 44 :1246–1252. [ PubMed ] [ Google Scholar ]
- Brodmann K. English translation by Laurence Garey of the German book, translator. London, UK: Smith-Gordon; 1909. Brodmann’s ’Localisation in the Cerebral Cortex. [ Google Scholar ]
- Clark RE, Manns JR, Squire LR. Classical conditioning, awareness, and brain systems. Trends Cogn Sci. 2002; 6 :524–531. [ PubMed ] [ Google Scholar ]
- Corkin S. Acquisition of motor skill after bilateral medial temporal lobe excision. Neuropsychologia. 1968; 6 :255–265. [ Google Scholar ]
- Corkin S. Lasting consequences of bilateral medial temporal lobectomy: Clinical course and experimental findings in H.M. Semin Neurol. 1984; 4 :249–259. [ Google Scholar ]
- Corkin S. What’s new with the amnesic patient H.M.? Nat Rev Neurosci. 2002; 3 :153–160. [ PubMed ] [ Google Scholar ]
- Corkin S. Permanent Present Tense: The Unforgettable Life of the Amnesic Patient, H. M. New York: Basic Books; 2013. p. 400. [ Google Scholar ]
- Corkin S, Amaral DG, Gonzalez RG, Johnson KA, Hyman BT. H. M.’s medial temporal lobe lesion: Findings from magnetic resonance imaging. J Neurosci. 1997; 17 :3964–3979. [ PMC free article ] [ PubMed ] [ Google Scholar ]
- Dale AM, Fischl B, Sereno MI. Cortical surface-based analysis. I. Segmentation and surface reconstruction. Neuroimage. 1999; 9 :179–194. [ PubMed ] [ Google Scholar ]
- Deoni SC. High-resolution T1 mapping of the brain at 3T with driven equilibrium single pulse observation of T1 with high-speed incorporation of RF field inhomogeneities (DESPOT1-HIFI) J Magn Reson Imaging. 2007; 26 :1106–1111. [ PubMed ] [ Google Scholar ]
- Deoni SC, Peters TM, Rutt BK. High-resolution T1 and T2 mapping of the brain in a clinically acceptable time with DESPOT1 and DESPOT2. Magn Reson Med. 2005; 53 :237–241. [ PubMed ] [ Google Scholar ]
- Deoni SC, Rutt BK, Peters TM. Rapid combined T1 and T2 mapping using gradient recalled acquisition in the steady state. Magn Reson Med. 2003; 49 :515–526. [ PubMed ] [ Google Scholar ]
- Ding SL, Van Hoesen GW. Borders, extent, and topography of human perirhinal cortex as revealed using multiple modern neuroanatomical and pathological markers. Hum Brain Mapp. 2010; 31 :1359–1379. [ PMC free article ] [ PubMed ] [ Google Scholar ]
- Ding SL, Van Hoesen GW, Cassell MD, Poremba A. Parcellation of human temporal polar cortex: A combined analysis of multiple cytoarchitectonic, chemoarchitectonic, and pathological markers. J Comp Neurol. 2009; 514 :595–623. [ PMC free article ] [ PubMed ] [ Google Scholar ]
- Eichenbaum H, Morton TH, Potter H, Corkin S. Selective olfactory deficits in case H.M. Brain. 1983; 106 :459–472. [ PubMed ] [ Google Scholar ]
- Erkinjuntti T. Vascular cognitive deterioration and stroke. Cerebrovasc Dis. 2007; 24 (Suppl 1):189–194. [ PubMed ] [ Google Scholar ]
- Fazekas F, Kleinert R, Offenbacher H, Schmidt R, Kleinert G, Payer F, Radner H, Lechner H. Pathologic correlates of incidental MRI white matter signal hyperintensities. Neurology. 1993; 43 :1683–1689. [ PubMed ] [ Google Scholar ]
- Fischl B, Salat DH, Busa E, Albert M, Dieterich M, Haselgrove C, van der Kouwe A, Killiany R, Kennedy D, Klaveness S, et al. Whole brain segmentation: Automated labeling of neuroanatomical structures in the human brain. Neuron. 2002; 33 :341–355. [ PubMed ] [ Google Scholar ]
- Fischl B, Salat DH, van der Kouwe AJ, Makris N, Segonne F, Quinn BT, Dale AM. Sequence-independent segmentation of magnetic resonance images. Neuroimage. 2004; 23 (Suppl 1):S69–S84. [ PubMed ] [ Google Scholar ]
- Freed DM, Corkin S. Rate of forgetting in H.M.: 6-month recognition. Behav Neurosci. 1988; 102 :823–827. [ PubMed ] [ Google Scholar ]
- Freed DM, Corkin S, Cohen NJ. Forgetting in H.M.: A second look. Neuropsychologia. 1987; 25 :461–471. [ PubMed ] [ Google Scholar ]
- Gabrieli JD, Cohen NJ, Corkin S. The impaired learning of semantic knowledge following bilateral medial temporal-lobe resection. Brain Cogn. 1988; 7 :157–177. [ PubMed ] [ Google Scholar ]
- Gabrieli JD, Milberg W, Keane MM, Corkin S. Intact priming of patterns despite impaired memory. Neuropsychologia. 1990; 28 :417–427. [ PubMed ] [ Google Scholar ]
- Gabrieli JD, Keane MM, Stanger BZ, Kjelgaard MM, Corkin S, Growdon JH. Dissociations among structural-perceptual, lexical-semantic, and event-fact memory systems in Alzheimer, amnesic, and normal subjects. Cortex. 1994; 30 :75–103. [ PubMed ] [ Google Scholar ]
- Gabrieli JD, McGlinchey-Berroth R, Carrillo MC, Gluck MA, Cermak LS, Disterhoft JF. Intact delay-eyeblink classical conditioning in amnesia. Behav Neurosci. 1995; 109 :819–827. [ PubMed ] [ Google Scholar ]
- Gaffan D. What is a memory system? Horel’s critique revisited. Behav Brain Res. 2001; 127 :5–11. [ PubMed ] [ Google Scholar ]
- Gaffan D, Parker A, Easton A. Dense amnesia in the monkey after transection of fornix, amygdala and anterior temporal stem. Neuropsychologia. 2001; 39 :51–70. [ PubMed ] [ Google Scholar ]
- Gloor P. The Temporal Lobe. Oxford University Press; New York and Oxford: 1995. p. 1997. [ Google Scholar ]
- Hebb DO. The organization of behavior. New York, NY: Wiley; 1949. [ Google Scholar ]
- Hebben N, Corkin S, Eichenbaum H, Shedlack K. Diminished ability to interpret and report internal states after bilateral medial temporal resection: Case H.M. Behav Neurosci. 1985; 99 :1031–1039. [ PubMed ] [ Google Scholar ]
- Horel JA. The neuroanatomy of amnesia. A critique of the hippocampal memory hypothesis. Brain. 1978; 101 :403–445. [ PubMed ] [ Google Scholar ]
- Insausti R, Tunon T, Sobreviela T, Insausti AM, Gonzalo LM. The human entorhinal cortex: A cytoarchitectonic analysis. J Comp Neurol. 1995; 355 :171–198. [ PubMed ] [ Google Scholar ]
- Insausti R, Juottonen K, Soininen H, Insausti AM, Partanen K, Vainio P, Laakso MP, Pitkanen A. MR volumetric analysis of the human entorhinal, perirhinal, and temporopolar cortices. AJNR Am J Neuroradiol. 1998; 19 :659–671. [ PMC free article ] [ PubMed ] [ Google Scholar ]
- Insausti R, Annese J, Amaral DG, Squire LR. Human amnesia and the medial temporal lobe illuminated by neuropsychological and neurohistological findings for patient E.P. Proc Natl Acad Sci USA. 2013; 110 :E1953–E1962. [ PMC free article ] [ PubMed ] [ Google Scholar ]
- James W. The Principles of Psychology: Dover Publications. New York: 1890. p. 1950. [ Google Scholar ]
- Keane MM, Gabrieli JD, Fennema AC, Growdon JH, Corkin S. Evidence for a dissociation between perceptual and conceptual priming in Alzheimer’s disease. Behav Neurosci. 1991; 105 :326–342. [ PubMed ] [ Google Scholar ]
- Keane MM, Gabrieli JD, Growdon JH, Corkin S. Priming in perceptual identification of pseudowords is normal in Alzheimer’s disease. Neuropsychologia. 1994; 32 :343–356. [ PubMed ] [ Google Scholar ]
- Keane MM, Gabrieli JD, Mapstone HC, Johnson KA, Corkin S. Double dissociation of memory capacities after bilateral occipital-lobe or medial temporal-lobe lesions. Brain. 1995; 118 :1129–1148. [ PubMed ] [ Google Scholar ]
- Kensinger EA, Ullman MT, Corkin S. Bilateral medial temporal lobe damage does not affect lexical or grammatical processing: Evidence from amnesic patient H.M. Hippocampus. 2001; 11 :347–360. [ PubMed ] [ Google Scholar ]
- Knopman D, Nissen MJ. Procedural learning is impaired in Huntington’s disease: Evidence from the serial reaction time task. Neuropsychologia. 1991; 29 :245–254. [ PubMed ] [ Google Scholar ]
- Lackner JR. Observations on the speech processing capabilities of an amnesic patient: Several aspects of H.M.’s language function. Neuropsychologia. 1974; 12 :199–207. [ PubMed ] [ Google Scholar ]
- Longstreth WT, Jr, Manolio TA, Arnold A, Burke GL, Bryan N, Jungreis CA, Enright PL, O’Leary D, Fried L. Clinical correlates of white matter findings on cranial magnetic resonance imaging of 3301 elderly people. The Cardiovascular Health Study. Stroke. 1996; 27 :1274–1282. [ PubMed ] [ Google Scholar ]
- Lorente de No R. Studies on the structure of the cerebral cortex II Continuation of the study of ammonic system. J Fur Psychol Neurol. 1934; 46 :113–177. [ Google Scholar ]
- Milner B. The memory defect in bilateral hippocampal lesions. Psychiatr Res Rep Am Psychiatr Assoc. 1959; 11 :43–58. [ PubMed ] [ Google Scholar ]
- Milner B. Les troubles de la memorie accompagnant des lesions hippocampiques bilaterales. In: Passouant P, editor. Physiologie de l’hippocampe. Paris: Centre National de la Recherche Scientifique; 1962. pp. 257–272. [ Google Scholar ]
- Milner B. Biology of Memory. New York: Academic Press, Inc; 1970. Memory and the medial temporal regions of the brain; pp. 29–50. [ Google Scholar ]
- Milner B, Corkin S, Teuber H-L. Further analysis of the hippocampal amnesic syndrome: 14-year follow-up study of H.M. Neuropsychologia. 1968; 6 :215–234. [ Google Scholar ]
- Mugler JP, III, Brookeman JR. Three-dimensional magnetization-prepared rapid gradient-echo imaging (3D MP RAGE) Magn Reson Med. 1990; 15 :152–157. [ PubMed ] [ Google Scholar ]
- Mugler JP, III, Bao S, Mulkern RV, Guttmann CR, Robertson RL, Jolesz FA, Brookeman JR. Optimized single-slab three-dimensional spin-echo MR imaging of the brain. Radiology. 2000; 216 :891–899. [ PubMed ] [ Google Scholar ]
- O’Kane G, Kensinger EA, Corkin S. Evidence for semantic learning in profound amnesia: An investigation with patient H.M. Hippocampus. 2004; 14 :417–425. [ PubMed ] [ Google Scholar ]
- Pascual-Leone A, Grafman J, Clark K, Stewart M, Massaquoi S, Lou JS, Hallett M. Procedural learning in Parkinson’s disease and cerebellar degeneration. Ann Neurol. 1993; 34 :594–602. [ PubMed ] [ Google Scholar ]
- Penfield W, Milner B. Memory deficit produced by bilateral lesions in the hippocampal zone. AMA Arch Neurol Psychiatry. 1958; 79 :475–497. [ PubMed ] [ Google Scholar ]
- Postle BR, Corkin S. Impaired word-stem completion priming but intact perceptual identification priming with novel words: Evidence from the amnesic patient H.M. Neuropsychologia. 1998; 36 :421–440. [ PubMed ] [ Google Scholar ]
- Price JL. Comparative aspects of amygdala connectivity. Ann N Y Acad Sci. 2003; 985 :50–58. [ PubMed ] [ Google Scholar ]
- Roemer PB, Edelstein WA, Hayes CE, Souza SP, Mueller OM. The NMR phased array. Magn Reson Med. 1990; 16 :192–225. [ PubMed ] [ Google Scholar ]
- Rosene DL, Van Hoesen GW. The hippocampal formation of the primate brain, a review of some comparative aspects of cytoarchitecture and connections. In: Jones EG, Peters A, editors. Cerebral Cortex. Further Aspects of Cortical Function, Including Hippocampus. New York: Plenum Press; 1987. pp. 345–456. [ Google Scholar ]
- Salat DH, Buckner RL, Snyder AZ, Greve DN, Desikan RS, Busa E, Morris JC, Dale AM, Fischl B. Thinning of the cerebral cortex in aging. Cereb Cortex. 2004; 14 :721–730. [ PubMed ] [ Google Scholar ]
- Salat DH, van der Kouwe AJ, Tuch DS, Quinn BT, Fischl B, Dale AM, Corkin S. Neuroimaging H.M.: A 10-year follow-up examination. Hippocampus. 2006; 16 :936–945. [ PubMed ] [ Google Scholar ]
- Sanes JN, Dimitrov B, Hallett M. Motor learning in patients with cerebellar dysfunction. Brain. 1990; 113 :103–120. [ PubMed ] [ Google Scholar ]
- Schmolck H, Kensinger EA, Corkin S, Squire LR. Semantic knowledge in patient H.M. other patients with bilateral medial and lateral temporal lobe lesions. Hippocampus. 2002; 12 :520–533. [ PubMed ] [ Google Scholar ]
- Scoville WB. The Limbic Lobe in Man. J Neurosurg. 1954; 11 :64–66. [ PubMed ] [ Google Scholar ]
- Scoville WB, Milner B. Loss of recent memory after bilateral hippocampal lesions. J Neurol Neurosurg Psychiatry. 1957; 20 :11–21. [ PMC free article ] [ PubMed ] [ Google Scholar ]
- Stefanacci L, Buffalo EA, Schmolck H, Squire LR. Profound amnesia after damage to the medial temporal lobe: A neuroanatomical and neuropsychological profile of patient E. P. J Neurosci. 2000; 20 :7024–7036. [ PMC free article ] [ PubMed ] [ Google Scholar ]
- Steinvorth S, Levine B, Corkin S. Medial temporal lobe structures are needed to re-experience remote autobiographical memories: Evidence from H.M. and W.R. Neuropsychologia. 2005; 43 :479–496. [ PubMed ] [ Google Scholar ]
- Thompson RF, Kim JJ. Memory systems in the brain and localization of a memory. Proc Natl Acad Sci USA. 1996; 93 :13438–13444. [ PMC free article ] [ PubMed ] [ Google Scholar ]
- Tovi M, Ericsson A. Measurements of T1 and T2 over time in formalin-fixed human whole-brain specimens. Acta Radiol. 1992; 33 :400–404. [ PubMed ] [ Google Scholar ]
- Van der Kouwe A, Benner T. Combined brain morphometry and skull imaging with FLUSTER. Sydney; Australia: 2008. [ Google Scholar ]
- van der Kouwe AJ, Benner T, Fischl B, Schmitt F, Salat DH, Harder M, Sorensen AG, Dale AM. On-line automatic slice positioning for brain MR imaging. Neuroimage. 2005; 27 :222–230. [ PubMed ] [ Google Scholar ]
- van der Kouwe AJ, Benner T, Dale AM. Real-time rigid body motion correction and shimming using cloverleaf navigators. Magn Reson Med. 2006; 56 :1019–1032. [ PubMed ] [ Google Scholar ]
- van der Kouwe AJ, Benner T, Salat DH, Fischl B. Brain morphometry with multiecho MPRAGE. Neuroimage. 2008; 40 :559–569. [ PMC free article ] [ PubMed ] [ Google Scholar ]
- Van Hoesen GW. Anatomy of the medial temporal lobe. Magnet Reson Imaging. 1995; 13 :1047–1055. [ PubMed ] [ Google Scholar ]
- Van Hoesen GW. Cortical feedforward and cortical feedback neural systems in Alzheimer’s disease. In: Hyman BT, Duychaerts C, Christen Y, editors. Connections, Cognition and Alzheimer’s Disease. Berlin, Heidelberg: Springer-Verlag; 1997. [ Google Scholar ]
- Van Hoesen GW, Pandya DN. Some connections of the entorhinal (area 28) and perirhinal (area 35) cortices of the rhesus monkey. III. Efferent connections. Brain Res. 1975; 95 :39–59. [ PubMed ] [ Google Scholar ]
- Van Hoesen GW, Pandya DN, Butters N. Cortical afferents to the entorhinal cortex of the Rhesus monkey. Science. 1972; 175 :1471–1473. [ PubMed ] [ Google Scholar ]
- Van Hoesen GW, Augustinack JC, Dierking J, Redman SJ, Thangavel R. The parahippocampal gyrus in Alzheimer’s disease. Clinical and preclinical neuroanatomical correlates. In: Scharfman W, Schwarcz, editors. The Parahippocampal Region, Implications for Neurological and Psychiatric Diseases. Ann N Y Acad Sci. Vol. 911. 2000. pp. 254–274. [ PubMed ] [ Google Scholar ]
- von Bonin G, Bailey P. The Neocortex of Macaca Mulatta. Urbana, IL: University of Illinois Press; 1947. [ Google Scholar ]
- von Economo C, Koskinas GN. Atlas of Cytoarchitectonics of the Adult Human Cerebral Cortex. Karger Publications; Berlin: 1925. [ Google Scholar ]
- Wechsler D. A standardized memory scale for clinical use. J Psychol. 1945; 19 :87–95. [ Google Scholar ]
- Weisz DJ, Harden DG, Xiang Z. Effects of amygdala lesions on reflex facilitation and conditioned response acquisition during nictitating membrane response conditioning in rabbit. Behav Neurosci. 1992; 106 :262–273. [ PubMed ] [ Google Scholar ]
- Wiggins GC, Triantafyllou C, Potthast A, Reykowski A, Nittka M, Wald LL. 32-channel 3 T receive-only phased-array head coil with soccer-ball element geometry. Magn Reson Med. 2006; 56 :216–223. [ PubMed ] [ Google Scholar ]
- Woodruff-Pak DS. Eyeblink classical conditioning in H.M.: Delay and trace paradigms. Behav Neurosci. 1993; 107 :911–925. [ PubMed ] [ Google Scholar ]
- Yarnykh VL. Actual flip-angle imaging in the pulsed steady state: A method for rapid three-dimensional mapping of the transmitted radiofrequency field. Magn Reson Med. 2007; 57 :192–200. [ PubMed ] [ Google Scholar ]
- Yonelinas AP, Jacoby LL. The process-dissociation approach two decades later: Convergence, boundary conditions, and new directions. Mem Cognit. 2012; 40 :663–680. [ PubMed ] [ Google Scholar ]
- Young VG, Halliday GM, Kril JJ. Neuropathologic correlates of white matter hyperintensities. Neurology. 2008; 71 :804–811. [ PubMed ] [ Google Scholar ]
- Zola-Morgan S, Squire LR. Memory impairment in monkeys following lesions limited to the hippocampus. Behav Neurosci. 1986; 100 :155–160. [ PubMed ] [ Google Scholar ]
- Zola-Morgan S, Squire LR, Mishkin M. The neuroanatomy of amnesia: Amygdala-hippocampus versus temporal stem. Science. 1982; 218 :1337–1339. [ PubMed ] [ Google Scholar ]
- Future Leaders in Neurology
- ACTRIMS 2024
- Treating NMOSD: What's Next?

- Conferences
- Publications
Patient H.M. & Neurology Research: Ethical or Not?
Although tremendous advances in research and medical ethics have been made since the mid-20th century, questions continue.
The author of a recently published book questions ethics of research involving one of the best-known neurology patients, evoking a passionate response from both the public and the research community.
The positive impact of medical research on the quality of life today is undoubted. Along with the victories, medical research had its failures, such as devastating consequences of early-day psychosurgery, but lessons from these early failures contributed to success of modern research just as much. The case of Henry Molaison, formerly known as Patient H. M., is one of such therapeutic failures that taught us so much. Breakthroughs in understanding of memory aside, an important lesson learned from this and similar cases was that of ethics. Although tremendous advances in research and medical ethics have been made since the mid-20th century, it continues to be questioned. Most recently, the issue came up in Patient H.M.: A Story of Memory, Madness, and Family Secrets , a book by Luke Dittrich. 1
Briefly, in a fragment of his book published in The New York Times Magazine , Dittrich suggests that Suzanne Corkin, PhD, a researcher who dedicated her life to studying Patient H. M., destroyed research data, opposed publication that could question the validity of her work, and violated research ethics principles. 2 The fragment and, shortly after, the book evoked a range of responses from the public, research community, and the Massachusetts Institute of Technology, where Corkin conducted her research.
The public found Corkin’s actions, as described by Dittrich, disturbing, judging by the comments on The New York Times website such as “Dr. Corkin comes across as evil!” Many were convinced by Dittrich’s story, which he, as a journalist, so skillfully tells. (I was unable to confirm whether Dittrich has any medical or research background.) In a follow-up interview to The New York Times , Dittrich shares his reasons for telling the story: “I believe Henry’s story is important … because of what his case can teach us about our sometimes ruthless pursuit of knowledge.” 3
Even if we assume for a second that Corkin indeed pursued her research interests ruthlessly, this lesson has been learned many times over, and multiple measures are now in place to minimize the risk to research participants. Thus, Dittrich’s motives for telling his version of this story are unclear to me, as is its educational value. In fact, his mention of “researchers who’d built their careers on [Henry] and who had an interest in presenting his story in a particular way” made me wonder about Dittrich’s interest in telling the story in the way he does.
Unlike the public, I was not disturbed by Corkin’s actions because I am familiar with research practices. I found her actions not only acceptable but perhaps even appropriate, and my opinion echoed that of the research community’s. 4 As I am not currently involved in research and do not represent interests of any research institution, my opinion lacks bias the public might suspect in opinions of researchers.
What I did find disturbing was the fact that the book was published shortly after Corkin’s death, when she could no longer defend her work and remediate the damage the book did to the public perception of research. I was very pleased to see the research community take on this role by sending an open letter to The New York Times . 5 As long as journalists with little knowledge of research practices feel the need to tell their stories, researchers must continue to tell theirs to help the public to form an educated, unbiased opinion.
References:
1. Dittrich L. Patient H.M.: a Story of Memory, Madness, and Family Secrets. New York: Random House; 2016.
2. Dittrich L. A brain that could not remember. The New York Times Web site. http://www.nytimes.com/2016/08/07/magazine/the-brain-that-couldnt-remember.html?_r=1 Accessed October 16, 2016.
3. Carey B. A Brain Surgeon’s Legacy Through a Grandson’s Eyes. The New York Times Web site. http://www.nytimes.com/2016/08/09/health/brain-patient-hm-book-dittrich.html Accessed October 16, 2016.
4. Hurley D. New allegations in book about patient HM kick up controversy on medical, scientific ethics . Neurology Today. 2016;16(19):48-50.
5. International Community of Scientists. Letter to the Editor of the New York Times Magazine. The MIT Department of Brain and Cognitive Sciences Web site. https://bcs.mit.edu/news-events/news/letter-editor-new-york-times-magazine Accessed October 16, 2016.
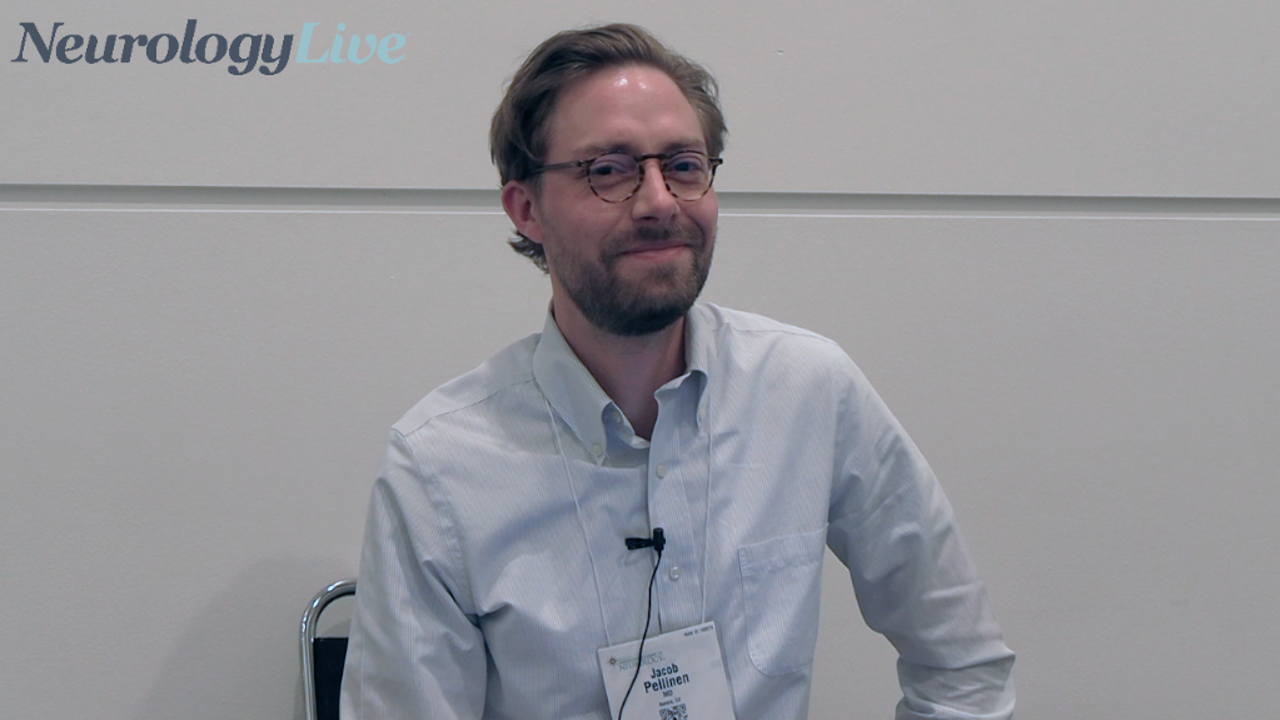
This Week on NeurologyLive® — April 15, 2024
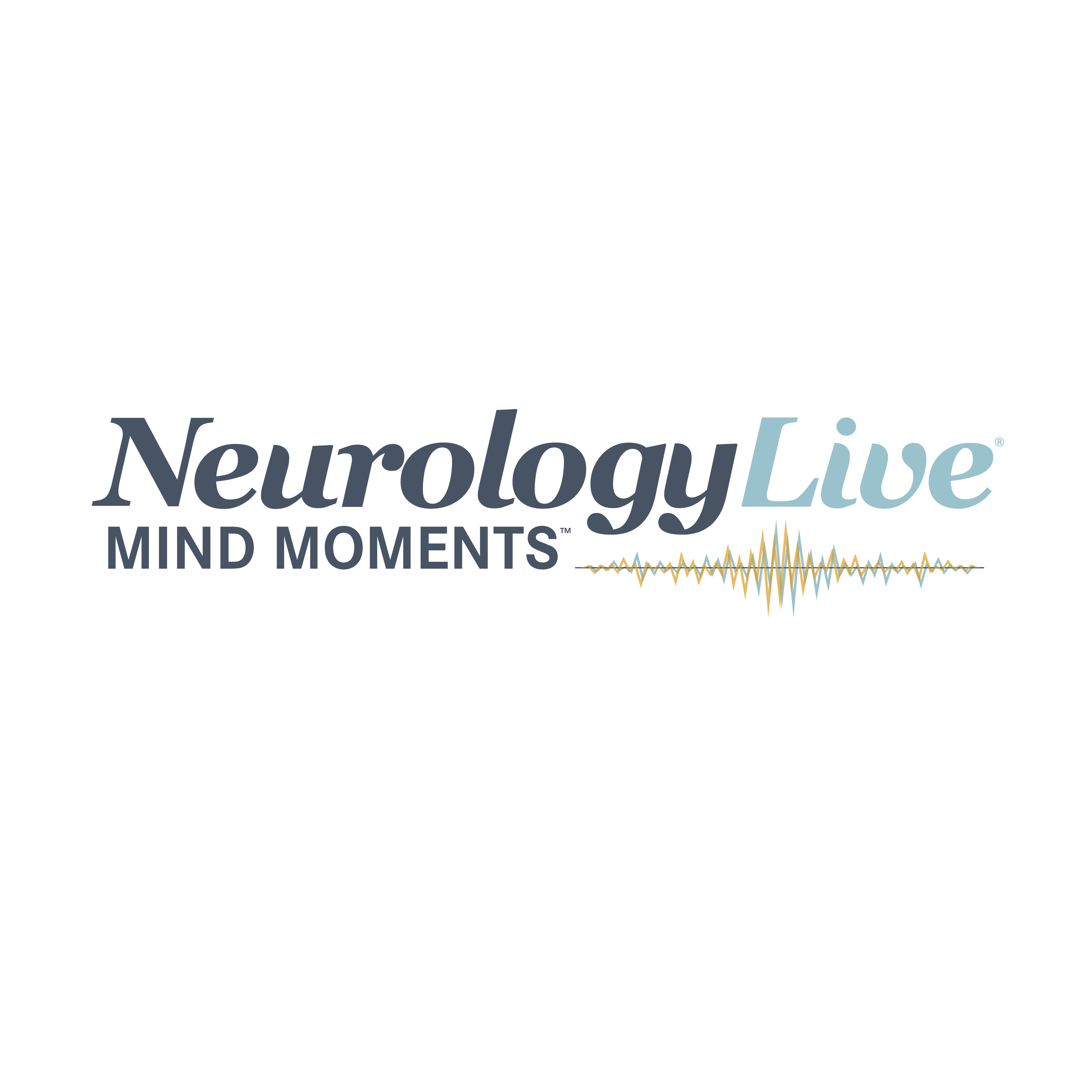
Episode 107: Revealing Mechanisms of Sudden Unexplained Death in Toddlers
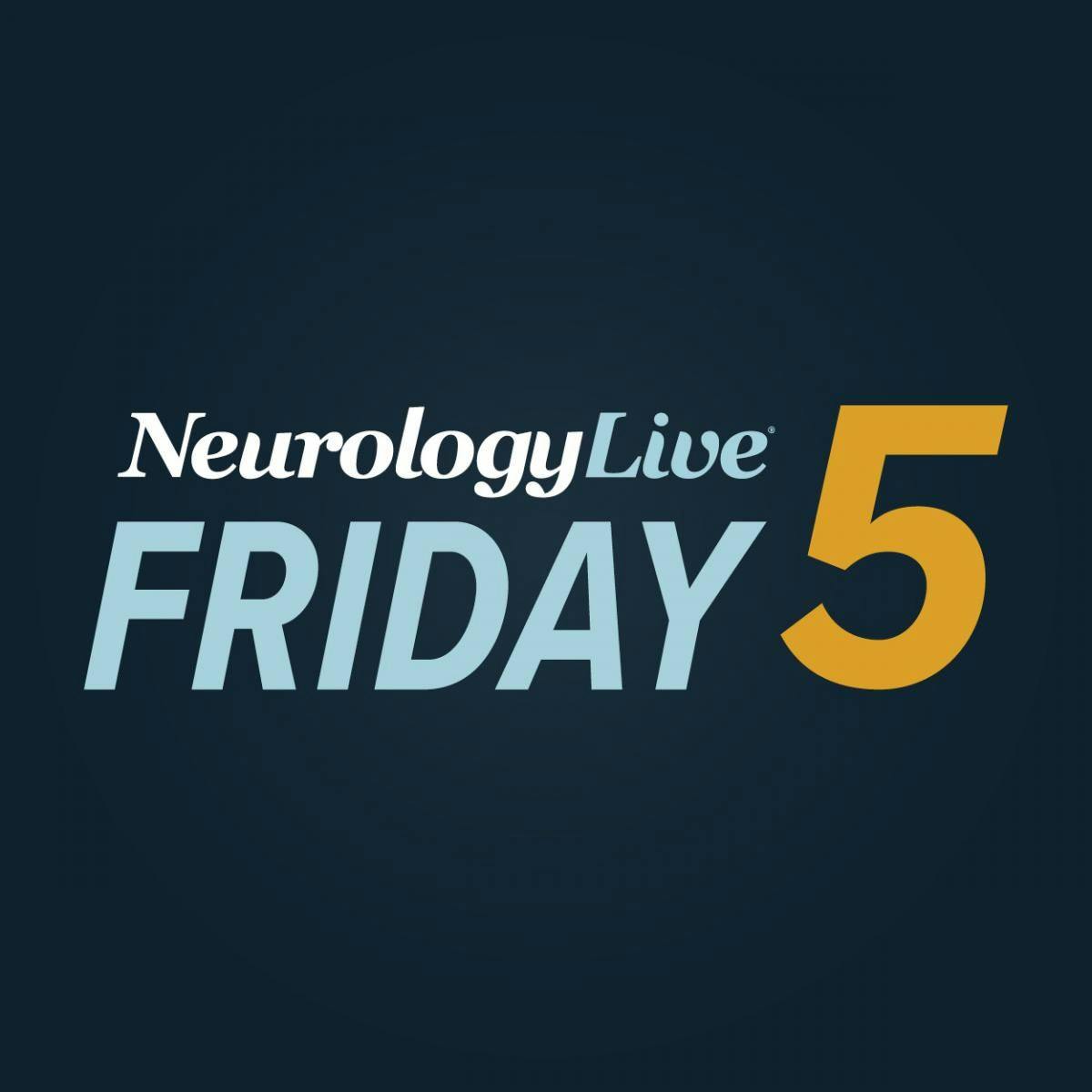
NeurologyLive® Friday 5 — April 12, 2024
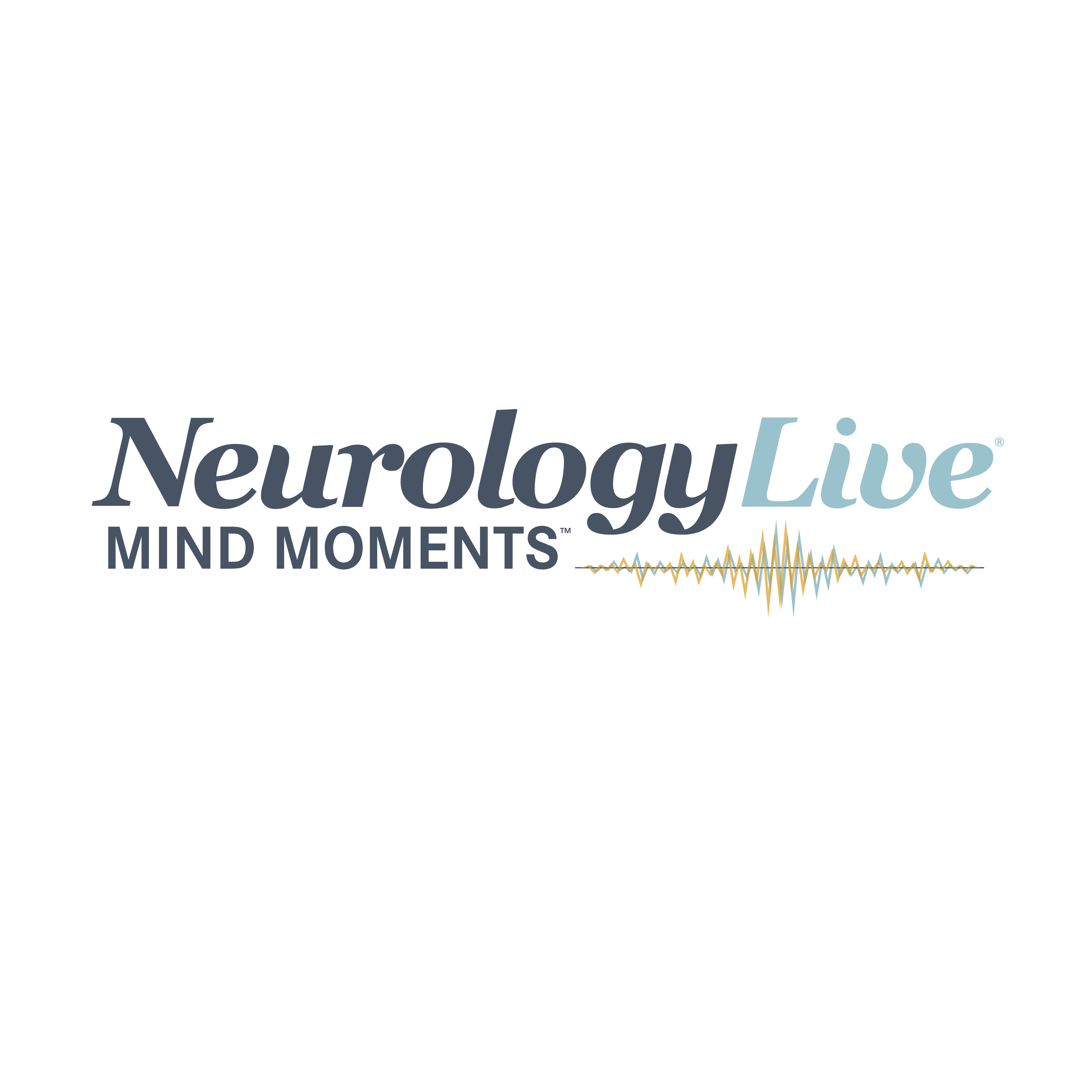
Episode 105: Recapping Conversations in Neurology From 2023
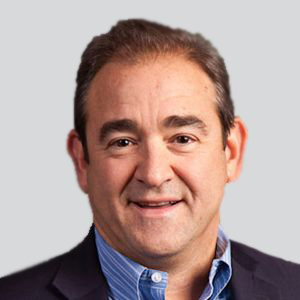
Marinus Reports Several Positive Trial Updates on Antiseizure Medication Ganaxolone
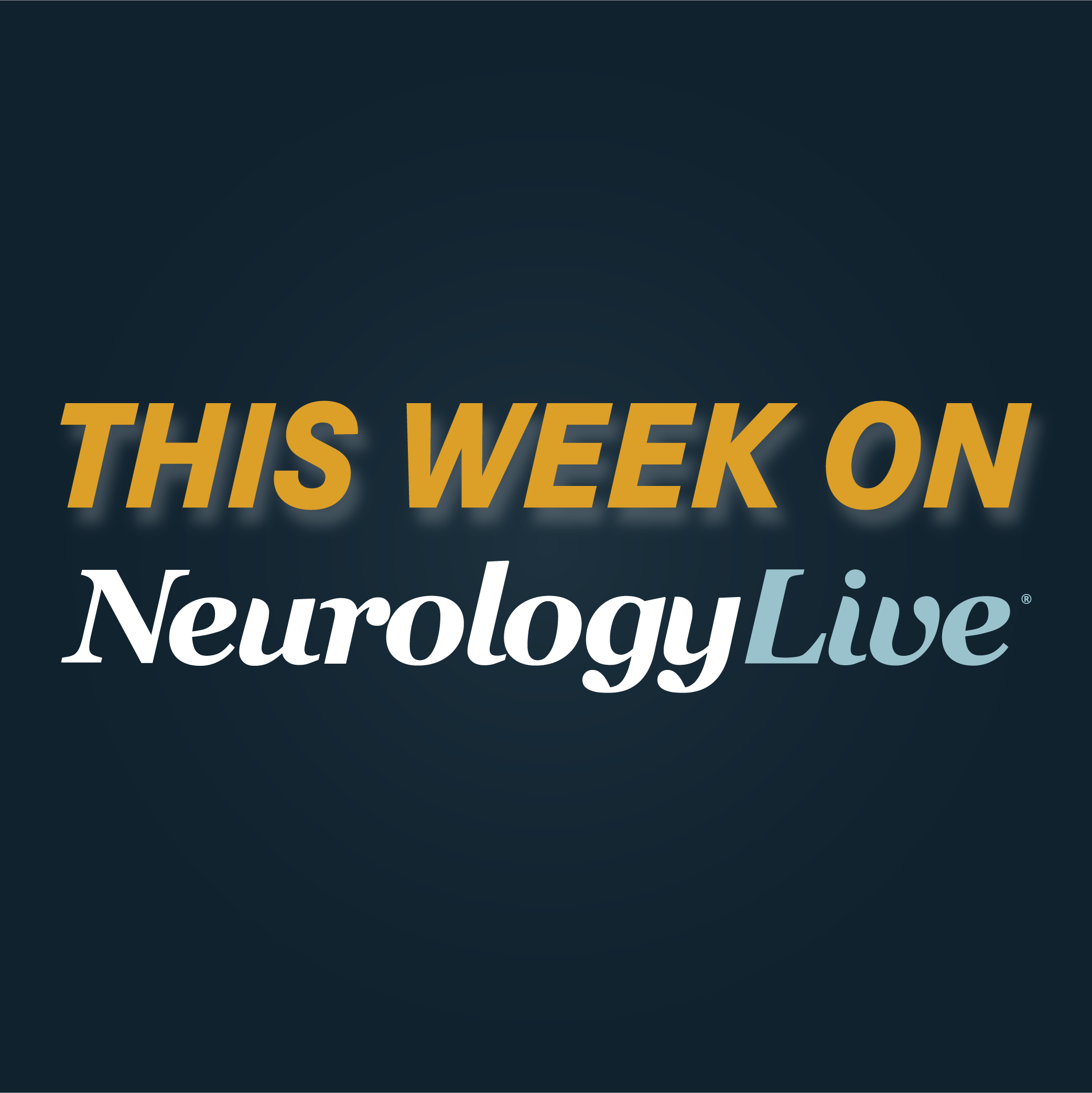
This Week on NeurologyLive® — April 8, 2024
2 Commerce Drive Cranbury, NJ 08512
609-716-7777

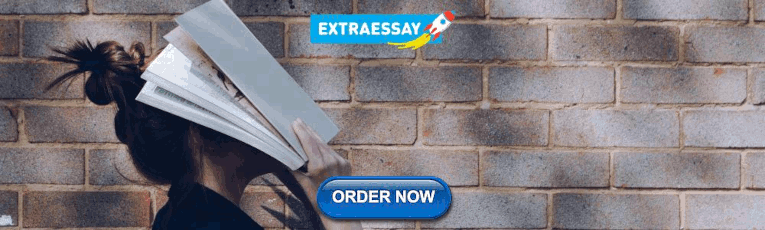
IMAGES
VIDEO
COMMENTS
Henry Gustav Molaison, known as Patient H.M., is a landmark case study in psychology. After a surgery to alleviate severe epilepsy, which removed large portions of his hippocampus, he was left with anterograde amnesia, unable to form new explicit memories, thus offering crucial insights into the role of the hippocampus in memory formation.
Henry died on December 2, 2008, at the age of 82. Until then, he was known to the world only as "HM," but on his death his name was revealed. A man with no memory is vulnerable, and his initials ...
Henry Molaison (or HM was he was known when he was alive) is one of the most important case studies in psychology. HM's case study is one of the most famous and important case studies in psychology, especially in cognitive psychology. It was the source of groundbreaking new knowledge on the role of the hippocampus in memory.
The Curious Case of Patient H.M. On September 1, 1953, time stopped for Henry Molaison. For roughly 10 years, the 27-year-old had suffered severe seizures. By 1953, they were so debilitating he could no longer hold down his job as a motor winder on an assembly line. On September 1, Molaison allowed surgeons to remove a thumb-sized section of ...
The story of Henry Molaison is a sad one. Known as Patient H.M. to the medical community, he lost the ability to create memories after he underwent a lobotomy to treat his seizures. He did earn a ...
The third and most studied of these three cases is patient Henry Molaison (H.M.). H.M. suffered a dense and persistent anterograde amnesia following bilateral medial temporal lobectomy in 1953 to treat intractable epilepsy (Scoville & Milner, 1957). His scientific fame derives from the dramatic demonstration of the critical role that the mesial ...
H.M. was the pseudonym of Henry Molaison, a man who was destined to change the way we think about the brain. Permanent Present Tense: The Unforgettable Life of the Amnesic Patient H.M. is a touching, comprehensive view of his life through the eyes of a researcher who also, in a sense, became part of his family. The prologue opens with a conversation between the author, Suzanne Corkin, and ...
Henry Gustav Molaison (February 26, 1926 - December 2, 2008), known widely as H.M., was an American who had a bilateral medial temporal lobectomy to surgically resect the anterior two thirds of his hippocampi, parahippocampal cortices, entorhinal cortices, piriform cortices, and amygdalae in an attempt to cure his epilepsy.Although the surgery was partially successful in controlling his ...
H.M. was likely the most studied individual in the history of neuroscience. Interest in the case can be attributed to a number of factors, including the unusual purity and severity of the memory impairment, its stability, its well-described anatomical basis, and H.M.'s willingness to be studied.
Aug. 24, 2016. For nearly six decades, Henry Molaison was known only by his initials, H.M., a brutally fitting detail for a man whose identity had more or less been erased. On Aug. 25, 1953, a ...
Studies on Patient H.M. showed that bilateral resection of the hippocampus results in impaired consolidation of long-term memory. Annese et al.create a digital map of Henry Molaison's brain and ...
Henry Molaison, also known as H.M. or Henry M., was born on February 26, 1926 to middle-class parents in Manchester, CT. When he was 9-years old, he was involved in a bicycle accident, sustaining a laceration of the left supra-orbital region with an approximate 5-min loss of consciousness. Shortly thereafter, around the age of 10, he began ...
Claudia Hammond revisits the classic case studies that have advanced psychological research. ... For 55 years, until he died in December 2008 at the age of 82, HM - or Henry Molaison, as he was ...
In the mid-1950's the surgeon Dr. Scoville was mortified to discover that his operation had ruined so much of H.M.'s memory, even though it did relieve H.M.'s epilepsy and probably saved his life ...
Henry Molaison, also known as H.M. or Henry M., was born on February 26, 1926 to middle-class parents in Manchester, CT. When hewas 9-years old,hewas involved in a bicycle accident, sustaining a laceration of the left supra-orbital region with an approximate 5-min loss of consciousness. Shortly thereafter, around the age of 10, he
This section provides a historical perspective and contributions from one of the most studied patients in neuroscience, Henry Molaison (1926-2008), known as H.M during his life to protect his privacy.
On Tuesday evening at 5:05, Henry Gustav Molaison known worldwide only as H. M., to protect his privacy died of respiratory failure at a nursing home in Windsor Locks, Conn. His death was ...
Current Study. H.M. died on December 2, 2008. That night, we conducted a wide variety of in situ MRI scans in a 3 T scanner at the Mass General Athinoula A. Martinos Center for Biomedical Imaging. The next morning, H.M.'s body was transported to the Mass General Morgue where Matthew Frosch, Director of the Neuropathology Unit, performed an ...
Henry Gustav Molaison. The case of Henry Gustav Molaison, who is often referred to as patient H.M. in psychology studies, aimed to cure H.M.'s epilepsy through brain surgery. Due to a bicycle ...
The case of Henry Molaison, formerly known as Patient H. M., is one of such therapeutic failures that taught us so much. Breakthroughs in understanding of memory aside, an important lesson learned from this and similar cases was that of ethics. Although tremendous advances in research and medical ethics have been made since the mid-20th century ...
H.M. is a very famous patient who suffered memory loss and had an absolute transformative effect on our understanding of memory and the brain. He had a very severe case of epilepsy that could not be treated pharmacologically with medical treatments, drug treatments. And so they performed surgery on him when he was in his late 20s, and removed ...
In 1953, Henry Molaison (known in the academic literature simply as "Patient HM") agreed to undergo an experimental procedure where a part of his brain locat...
Given the effect of urbanization on land use and the allocation and implementation of urban green spaces, this paper attempts to analyze the distribution and accessibility of public parks in India's Bengaluru city (previously known as Bangalore). Availability, accessibility, and utilization—the key measures of Urban Green Spaces (UGS)—are mostly used in health research and policy and are ...