- Open access
- Published: 02 April 2020
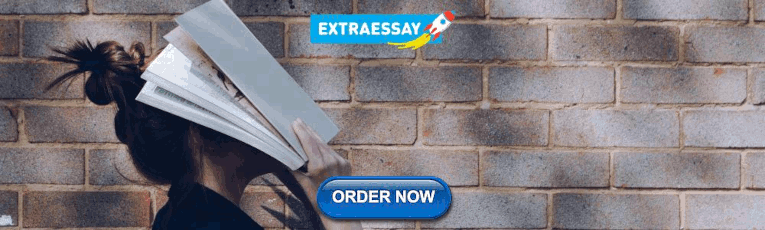
Growing a circular economy with fungal biotechnology: a white paper
- Vera Meyer ORCID: orcid.org/0000-0002-2298-2258 1 ,
- Evelina Y. Basenko ORCID: orcid.org/0000-0001-8611-5447 2 ,
- J. Philipp Benz ORCID: orcid.org/0000-0001-5361-4514 3 ,
- Gerhard H. Braus ORCID: orcid.org/0000-0002-3117-5626 4 ,
- Mark X. Caddick ORCID: orcid.org/0000-0002-5489-6557 2 ,
- Michael Csukai ORCID: orcid.org/0000-0003-0615-7802 5 ,
- Ronald P. de Vries ORCID: orcid.org/0000-0002-4363-1123 6 ,
- Drew Endy ORCID: orcid.org/0000-0001-6952-8098 7 ,
- Jens C. Frisvad ORCID: orcid.org/0000-0002-0573-4340 8 ,
- Nina Gunde-Cimerman ORCID: orcid.org/0000-0002-9464-3263 9 ,
- Thomas Haarmann ORCID: orcid.org/0000-0001-6033-8140 10 ,
- Yitzhak Hadar ORCID: orcid.org/0000-0001-7510-7847 11 ,
- Kim Hansen ORCID: orcid.org/0000-0002-2670-4677 12 ,
- Robert I. Johnson 13 ,
- Nancy P. Keller ORCID: orcid.org/0000-0002-4386-9473 14 ,
- Nada Kraševec ORCID: orcid.org/0000-0002-0925-4272 15 ,
- Uffe H. Mortensen ORCID: orcid.org/0000-0002-7794-7273 8 ,
- Rolando Perez 7 ,
- Arthur F. J. Ram ORCID: orcid.org/0000-0002-2487-8016 16 ,
- Eric Record ORCID: orcid.org/0000-0002-7545-9997 17 ,
- Phil Ross 18 ,
- Volha Shapaval ORCID: orcid.org/0000-0003-2674-1328 19 ,
- Charlotte Steiniger ORCID: orcid.org/0000-0001-5329-2391 1 ,
- Hans van den Brink 20 ,
- Jolanda van Munster ORCID: orcid.org/0000-0003-0412-7001 21 ,
- Oded Yarden ORCID: orcid.org/0000-0002-4722-535X 11 &
- Han A. B. Wösten ORCID: orcid.org/0000-0002-0399-3648 22
Fungal Biology and Biotechnology volume 7 , Article number: 5 ( 2020 ) Cite this article
59k Accesses
213 Citations
149 Altmetric
Metrics details
Fungi have the ability to transform organic materials into a rich and diverse set of useful products and provide distinct opportunities for tackling the urgent challenges before all humans. Fungal biotechnology can advance the transition from our petroleum-based economy into a bio-based circular economy and has the ability to sustainably produce resilient sources of food, feed, chemicals, fuels, textiles, and materials for construction, automotive and transportation industries, for furniture and beyond. Fungal biotechnology offers solutions for securing, stabilizing and enhancing the food supply for a growing human population, while simultaneously lowering greenhouse gas emissions. Fungal biotechnology has, thus, the potential to make a significant contribution to climate change mitigation and meeting the United Nation’s sustainable development goals through the rational improvement of new and established fungal cell factories. The White Paper presented here is the result of the 2nd Think Tank meeting held by the EUROFUNG consortium in Berlin in October 2019. This paper highlights discussions on current opportunities and research challenges in fungal biotechnology and aims to inform scientists, educators, the general public, industrial stakeholders and policymakers about the current fungal biotech revolution.
Introduction
The term ‘biotechnology’ was coined by the Hungarian Karl Ereky [ 1 ] in 1919, the same year that Pfizer became the first company to commercialise a product manufactured by the controlled fermentation of a mould. The product Pfizer made was citric acid for commercial use as a flavouring agent, acidifier and chelating agent in food, beverages, and the pharma and chemical industries. It was produced biotechnologically with the mould Aspergillus niger [ 2 ], and 100 years later, citric acid is still produced with this filamentous fungus and has formed a fast growing multibillion Euro market for convenience foods and beverages [ 2 , 3 ]. Many organic acids, enzymes, life-saving antibiotics and drugs are produced by filamentous fungi, and a lot of our foods and beverages would not exist at all without their fermentative capacities [ 4 ]. It is undisputed that filamentous fungal-based biotechnology is of pivotal importance to our daily lives. Many companies around the globe are leveraging the power of filamentous fungi, with major players in Europe that include: AB Enzymes, BASF, Bayer, Chr. Hansen, Dyadic International, DSM, DuPont, Kerry Group, Marlow Foods, Novozymes, Puratos, Syngenta and Roal Oy.
In a more recent endeavour, biologists, chemists, bioinformaticians, bioengineers, process engineers and material scientists have collaborated to turn by-products and waste from agriculture and forestry into composite materials with the help of mushroom-forming fungi. The vision offered by this group is disruptive: soon, we will sit on mushroom-created furniture, we will live in houses made from fungal hyphae, plastics and insulation materials in cars, trains, planes and spacecrafts will be composed of fungal composites and many of our textiles will be derived from fungi. In the not so distant future, new fungal-based products will be introduced into the market that offer similar or superior characteristics compared to classic petroleum-derived products, with a reduced or even negative carbon footprint and full biodegradability. Another vision is not so far-fetched at all: products made with animal leather will be superseded in quality and price by those made with pure fungal mycelium. Such replacements will have substantial implications for the livestock, textile and fashion industries.
Fungi growing as a yeast morphology have impacted society for millennia and have been instrumental for the production of bread, beer and wine. However, fungal species capable of the filamentous growth form, which are the focus of this White Paper, have additional beneficial properties such as the productions of a diverse array of metabolites, enzymes and materials. Indeed, Scientific American stated in 2019 that: “The mycelium revolution is upon us” [ 5 ]. A 100 years after the birth of fungal biotechnology, this platform technology is undergoing a renaissance by providing sustainable solutions for diverse industries and markets [ 6 ]. In the following, we will summarize current and anticipated products made from moulds and mushrooms and related new avenues of research. We will also highlight recommendations discussed during the EUROFUNG 2nd Think Tank consortium for ways to drive innovation in this renaissance through strategic and infrastructural measures for basic and applied science on filamentous fungi.
The lifestyle of filamentous fungi—moulds and mushrooms
The life of a filamentous fungus usually starts with a spore, which has a diameter of only a few microns (µm) (Fig. 1 ). The spore starts to swell in a humid and nutrient-rich environment and germinates. A germ tube is formed that elongates to eventually form a thread-like, filamentous cell, called a hypha. After the hypha grows and elongates for some time, it forms a network of interconnected hyphal threads called a mycelium. When nutrients become limited in the substrate within which the mycelium lives, the mycelium starts to explore the air and space in order to form reproductive structures. Ascomycetes (moulds) can form conidiophores that produce asexual spores at their ends and/or fruiting bodies that produce sexual spores inside them. Basidiomycetes are known for their ability to form fruiting bodies to generate sexual spores. Some of these fruiting bodies are colloquially called mushrooms. These fruiting bodies consist of mycelia which are more densely packed and different in their composition compared to the rather loose substrate mycelia, which forms three-dimensional net-like structures resembling the global system of interconnected computer networks.
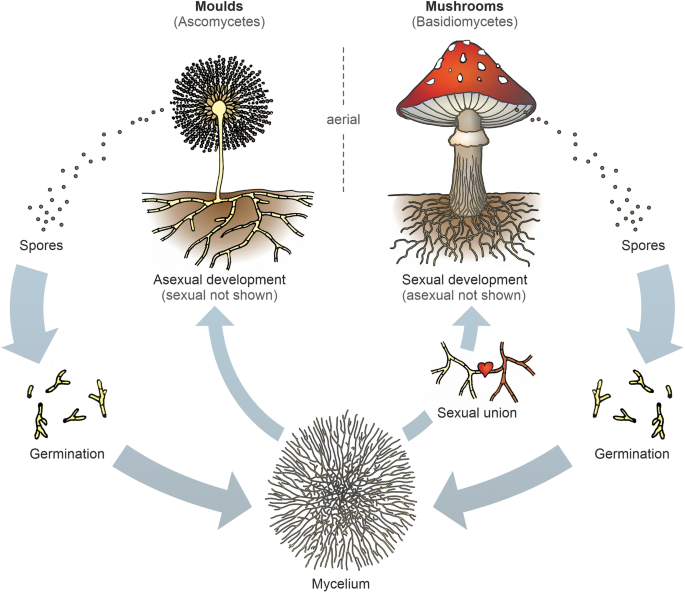
The fungal life cycle
Filamentous fungi are both invisible and visible. The diameter of fungal hyphae range from 2 to 10 µm, and a fungal mycelium consists of a network of mm- to cm-long hypha. The mycelia of mushroom-forming fungi can colonise large surface areas, as illustrated by an individual of the honey mushroom Armillaria bulbosa (common name honey mushroom in English and Hallimasch in German) which has colonised > 1000 hectares of forest soil, making it the largest and oldest organism on Earth [ 7 ]. Mycelia of mushroom-forming fungi can also be grown on various by-products and waste streams from forestry and agriculture. The efficiency of colonisation and biomass formation is determined by the composition and physical properties of the substrate, the environmental growth conditions (temperature, humidity and pH) and the genetic make-up of the fungus. A strain of Schizophyllum commune (common name split gill), for instance, in which two regulatory genes for mushroom formation are inactivated, produces threefold more biomass of fungal tissue when compared to the parental strain [ 8 ].
Filamentous fungi have evolved to become superbly efficient decomposers and have developed the ability to feed on and break down organic matter and polymeric substances [ 9 ]. Polysaccharides from plant biomass make up most of the biomass on Earth (450 out of a total of 550 gigatons of carbon; [ 10 ]) and represent the major carbon sources that drives fungal growth. In order to digest, humans must first ingest. Filamentous fungi first digest and then ingest. The fungal cells that infiltrate a substrate secrete enzymes, such as cellulases, amylases, pectinases, inulases, proteases and lipases, into the surrounding medium and hydrolyse (break down) plant polysaccharides (e.g. cellulose, starch, pectin, inulin), proteins and lipids. The degradation products of polysaccharides are monosaccharides, such as glucose, and oligosaccharides, which are subsequently taken up into the hyphae with the help of specific sugar transporters. This hydrolytic capacity for plant biomass, coupled with an extraordinary high secretion capacity for enzymes (up to 100 g/L are reported; [ 11 ]), forms the basis for the success of high-performance enzyme-producing cell factories, such as A. niger, A. oryzae, Trichoderma reesei and Thermothelomyces thermophilus (all being ascomycetes). Their enzyme products are exploited by a diverse array of major industries, including food and feed, detergent, pulp and paper, fuel, pharmaceutical and chemical (Fig. 2 ; [ 4 ]).
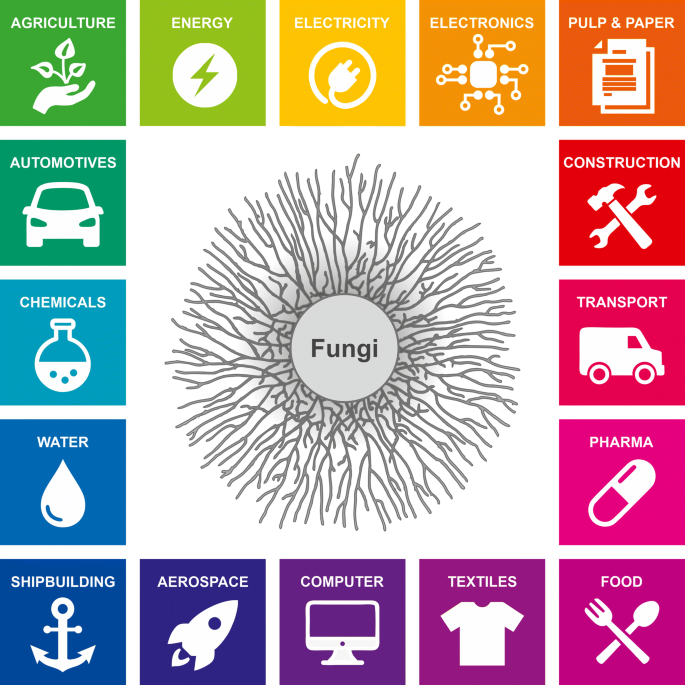
Industries profiting from the metabolic capacities of filamentous fungi
Notably, the predicted gene set for plant polysaccharide-degrading enzymes in these fungi is between 100 and 250, whereas only 30 can be found in the baker’s yeast Saccharomyces cerevisiae . The success of S. cerevisiae, which cannot grow on plant polymeric substances [ 12 ], or the bacterium Escherichia coli as platform organisms to produce biofuel (ethanol), jet fuel (terpenoids), fine and commodity chemicals is only guaranteed by the filamentous fungal cell factories mentioned above that provide S. cerevisiae and E. coli with simple carbon sources during the fermentation process. Hence, filamentous fungal cell factories play a central role in the sustainable production of biofuels and chemicals.
The product portfolio of filamentous fungi—an overview
The product range of filamentous fungi is not limited to citric acid and enzymes (Table 1 ). In fact, the natural metabolic capacities of filamentous fungi are extraordinarily diverse and unmatched in nature. Organic acids, chemicals, antibiotics and other drugs, proteins and enzymes, meat alternatives, vitamins, polyunsaturated fatty acids and even composite materials and vegan leathers are existing fungal products. Table 2 highlights some currently traded pharmaceuticals derived from filamentous fungi.
Note that T. thermophilus was formerly named Myceliophthora thermophila and that the P. chrysogenum strain used for penicillin production was recently reidentified as P. rubens [ 14 , 15 ]. While this review focuses on the Dikarya lineage, that is the ascomycetes and basidiomycetes, five species in this list are found in another lineage, the Mucoromycota.
Some examples of current developments with fungal cell factories that will help to consolidate bio-economies and human health are summarized as follows.
Aspergillus niger is currently being explored by academia as a potential producer of other organic acids beyond citric acid, such as itaconate and galactarate. Itaconate could replace petroleum-based polyacrylic acid, which is a precursor for the polymer industry (absorbent polymers, polyester resins, synthetic latex) and galactarate could replace the current petroleum-based polyethylene terephthalate (PET) used for plastic production [ 17 , 18 ].
A. oryzae , used to produce Asian food and beverages for over a 1000 years, has recently gained interest as a producer of malate, which has multiple applications in the food (acidulant, flavour enhancer), chemical (polyester resins) and pharmaceutical (acidulant) industries [ 19 ]. While several microbial cell factories from bacterial, yeast or filamentous fungal origin have been genetically engineered during the last few years to produce malate, A. oryzae is among the strains with the greatest yields [ 20 ].
A. terreus is exploited as a cell factory to produce two molecules: the organic acid itaconate, which is of interest for the polymer industry [ 21 ], and lovastatin, which is a cholesterol-lowering drug. Lovastatin has been marketed under the tradename Mevacor since the late 1980 s [ 22 ]. It, furthermore, serves as a starter molecule for manufacturing semisynthetic statins, such as simvastatin (trade name Zocor), which is the second leading statin on the market [ 23 ]. Current research is focused on re-routing lovastatin biosynthesis to one of its biosynthetic intermediates, monacolin J, which is the preferred precursor for Zocor synthesis [ 24 ]. Notably, a genetically engineered A. oryzae strain can reach monacolin J concentrations of about 5.5 g/L, which is considerably higher than has been achieved in heterologous hosts, such as S. cerevisiae (75 mg/L) or Pichia pastoris (renamed Komagataella phaffii ; 600 mg/L) [ 25 ].
P. chrysogenum ( P. rubens ) is an antibiotics producer and the main cell factory used to produce penicillin and semisynthetic derivatives, with production levels up to 55 g/L [ 26 ]. Notably, the biosynthetic route for penicillin has recently been genetically reprogrammed towards pravastatin, which constitutes an interesting alternative to Zocor for the pharma industry [ 27 ].
T. reesei and T. thermophilus (formally Myceliophthora thermophila ) are of commercial interest because of their ability to secrete cellulases and hemicellulases. These enzymes are key to converting lignocellulosic biomass into biofuel. Lignocellulose is composed of cellulose, hemicellulose and lignin and is a by-product of agriculture (e.g. straw, bagasse, corn stover) and forestry (e.g. sawdust). The thermostability of (hemi)cellulases from T. thermophilus are of special interest for current lignocellulose degradation processes, as high process temperatures are preferred in biorefineries to reduce the viscosity and increase the solubility of lignocellulosic biomass [ 28 ]. Genetically optimised hypersecreting strains have been established during the last two decades which achieve cellulase titres of 100 g/L [ 11 ]. These are the highest titres ever reported for protein secretion and exceed by 10–10,000-fold what can be achieved nowadays with bacterial, yeast or mammalian cell factories. Protein secretion titres in these cell factories are usually in the order of mg/L to only a few g/L [ 29 , 30 , 31 ]. Notably, T. thermophilus has been genetically reprogrammed to generate platform chemicals, such as fumarate, directly from renewable feedstocks to replace petroleum-based processes, which is of interest for the manufacturing of synthetic resins, biodegradable polymers (up to 17 g/L) and malate (up to 200 g/L) [ 32 , 33 ]. The fungus has also been optimised by Dyadic International as a producer for biologics, including vaccines, therapeutic enzymes, proteins and biosimilars [ 34 ].
Filamentous fungi as meat replacements
The use of fungi as a source of food predates recorded history [ 35 ]. These have been predominantly the mushrooms from supermarket shelves and foraging expeditions. The fruiting bodies of these fungi have a wide variety of tastes and textures and some of these are considered very meat-like, for example “Chicken of the Woods” ( Laetiporus sulphurous ) and “Beefsteak fungus” ( Fistulina hepatica ). There has recently been a move to create meat-like products from fungal mycelium grown in fermenters, rather than the solid fruiting bodies. This has allowed the introduction of ascomycetes, traditionally used as flavour modifiers in such foods as blue cheese, to enter the food chain as convincing meat substitutes. The longest established of these companies is Marlow Foods, using Fusarium venenatum under the trade name Quorn™, but several other companies have recently shown an interest in this area, including Mycorena ( A. oryzae ; [ 36 ]), Sustainable Bioproducts ( F. oxysporum ; [ 37 ]) and MycoTechnology, using the basidiomycete Lentinula edodes [ 38 ]. The hyphae of filamentous fungi, when aligned and organised, provide a structure that looks like and has the mouth feel of meat, particularly chicken, due to the similarity of fibre size (Fig. 3 ). The high amino acid and fibre content, and low saturated fat, combined with the high digestibility of fungal protein (see Additional file 1 : Table S1), make this an exceptionally healthy food [ 39 ].
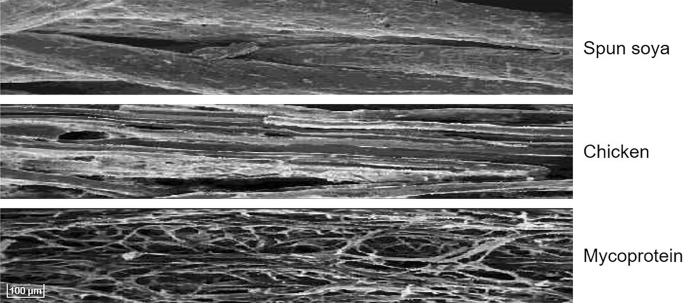
Unique textural attributes of Quorn™. Electron microscopic images of protein fibres from spun soya and chicken and hyphal filaments of F. venenatum . Bar, 100 µm
The fungi for food replacements are grown in fermenters on simple salts and glucose as the carbon source. Quorn™ uses air lift pressure cycle fermenters (Fig. 4 ). These are 30 m tall and have an operating volume of approximately 150 m 3 , which offer several operational and control benefits over conventional fermenters, including low shear stress allowing longer (and, therefore, higher quality) hyphae.
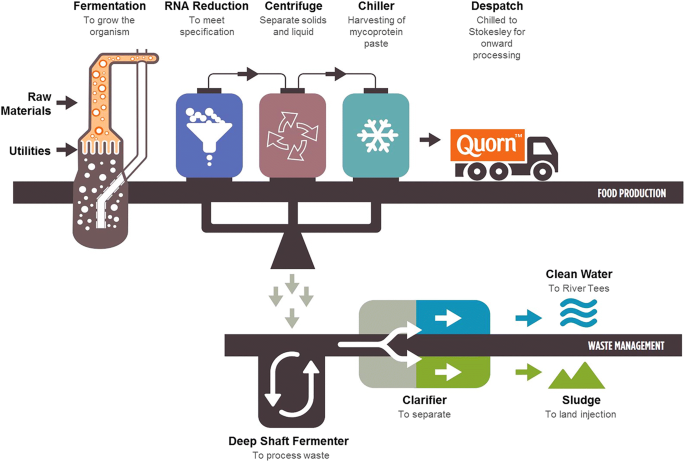
The Quorn™ fermentation process. A continuous supply of medium is fed into the fermenter and the broth is harvested simultaneously. The harvested broth is heated to a temperature that destroys proteases but leaves RNAses active, allowing the RNA content of the mycelium to be reduced to less than 2%, which is a regulatory requirement. Once the broth has been heat-treated, the mycelium is spun down to form a paste, which is mixed with binders and flavouring agents before being shaped, cooked and frozen. The supernatant from the paste is currently sent for treatment as wastewater, but active research at Marlow Foods is looking into how the 1.5% solids in the waste can be recovered as a food grade co-product
Glucose can be provided from several sources, currently by enzymatic digestion of starch from wheat or maize using the enzyme glucoamylase from A. niger , but lignocellulose is being considered for the future. As the protein from the original grain is retained for other uses and not fed to F. venenatum , the metric of the amount of protein per hectare, used to compare many animal proteins, is misleading when applied to fungi. The use of protein in animal feeds, whether animals or insects, acts to concentrate (with losses) what is present in the original, whereas fungi add protein to a protein-free feedstock. Furthermore, production of fungal protein is highly water-efficient in comparison to animal protein, requiring about one-tenth that of beef and half that of chicken. Quorn Foods was the first global meat alternative brand to achieve third party certification of its carbon footprint figures [ 40 ]. However, there is considerable potential to reduce the ecological impact of fermented F. venenatum further, and, as such, Marlow Foods are pursuing a research programme involving multiple external partners looking at water reduction, carbohydrate choice, fermenter optimisation, co-product valorisation and more.
Filamentous fungi as biomaterials
Fungi thrive by decomposing and consuming dead plants by breaking down the plant’s cellulose, lignin and other sugars, then rearranging these molecules into their own biomass to grow. Their cell wall is secured by chains of chitin and glucans, which, like cellulose and keratin, are naturally forming polymers found in the toughest of organic tissues. Chitin is the same ingredient that creates the durable and flexible exoskeletons of insects and shellfish [ 41 ]. During the colonisation of substrates, hyphae bind the organic particles together, while degrading them simultaneously. A composite material is obtained, consisting of a bulk of organic substrate bound together by the hyphal network, by inactivating the fungus before the substrate is degraded (e.g. by drying or by heat inactivation). Pure fungal materials are obtained by complete degradation of the substrate or removing the fungal skin from the substrate. Both pure and composite mycelium can be used for different applications [ 42 ].
Stopping fungal growth during colonisation of the substrate results in materials with properties similar to that of expanded polystyrene or other foams (Fig. 5 ; [ 43 , 44 ]). Such materials can be used as packaging material or for heat or acoustic insulation. The properties of both composite and pure mycelium materials are dependent on the substrate, the type of fungus, the growth conditions and post-processing. Heat pressing, for instance, improves the homogeneity, strength and stiffness of the mycelium composite material, shifting its performance from foam-like to cork- and wood-like (Fig. 5 ; [ 45 ]). A range of mycelium composite materials with different properties can be produced [ 46 ]. Future studies should focus on the coating of mycelium materials to reduce water uptake and volatile organic compound escape. The genetic engineering of strains, however, may make coating superfluous.
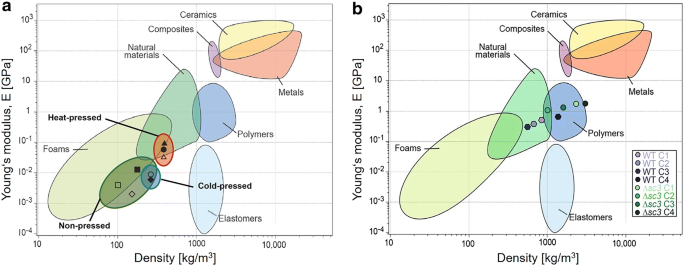
Material properties that can be achieved with fungal mycelia. a Mycelium composites. b Mycelium textiles. The pictures depicted are reproduced from [ 45 ], which has been published under a Creative Commons Attribution licence (CC BY, http://creativecommons.org/licenses/by/4.0/ )
Mushroom-based materials have the potential for uses in place of leather, textiles and some plastics. The strength of pure mycelium of a wild-type S. commune strain is similar to that of natural materials, such as wood and leather [ 47 ]. By contrast, a material can be obtained by deleting a gene that encodes a protein called hydrophobin, that pearls off water droplets from fruiting bodies, with a strength similar to that of thermoplastics. The difference in the material properties between these strains is explained by a better packing of the hyphae in the deletion strain, resulting in an increased density of the mycelium. In addition, the absence of the hydrophobin in this strain results in a mycelium that is no longer water-repellent, consequently, it absorbs more water and dries more slowly than the wild-type [ 47 ]. Thus, mycelium-based materials can provide desirable mechanical properties which can also be bio-performative, for example, by buffering water availability. Future studies, therefore, should focus on not only the mechanical but also bioactive properties, making mycelium a potential source of new innovative materials with advanced properties. Future studies should also reveal whether mycelia can be utilised to develop materials with properties similar to the material families of the elastomers, composites, metals and ceramics (Fig. 5 ).
The flexibility and aesthetics of mushroom materials were first appreciated by artists and designers who used them to grow living art works [ 48 , 49 ]. Similar to cement and plaster, mycelia will bind, harden and set into a variety of solidified configurations. These designers and artists soon discovered that materials grown from this dense living matrix could be used to make advanced composites, foams and performance plastics, and the commercial potential soon followed. Companies that are designing and engineering mycelium materials include MycoWorks [ 50 ], Ecovative Design [ 51 ], NEFFA [ 52 ] and MOGU [ 53 ], to name but a few. Research programmes at MycoWorks proved that fine mycelium materials can be grown into the texture of sheet urethanes, animal skins and foams, with surfaces that are velvety and fluffy, leathery and rubbery, or beetle-shell brittle and shiny. After a mycelium-based object has been grown, it can be cut, processed and machined like many other materials. Demand for these materials has been driven by concerns for stabilizing the price of supply chains into the future. One of the first markets for which fungal mycelium can provide a solution is the fashion industry, where there is both a consumer and market demand for alternatives to the animal skins and plastics used to make apparel. A sensual and strong material for luxury fashion has been developed by moulding the body of the mycelium into a sheet and processing it in many of the same ways as animal leather (Fig. 6 ).
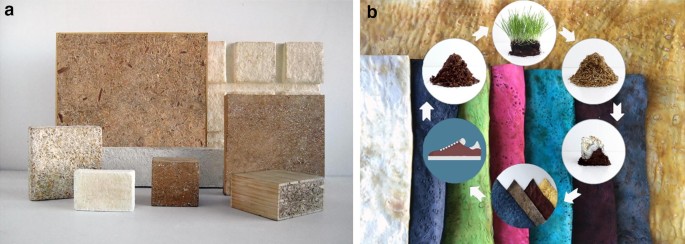
MycoWorks’ fungal analogues for composites and leather. a Analogues for synthetic wood composites and expanded polystyrene foams. Mushrooms are very sensitive to their surroundings, and it is possible by altering subtle factors to make their tissue express a range of variably determined physical characteristics. While these materials can be grown into building components for construction and interior architectures, they can also be grown with delicately tuneable qualities. The strength, durability and biodegradable nature of mushroom-based materials suggest many ways in which fungi may be used. When the material is processed with traditional industrial wrapping and laminating equipment, it is possible to create functional materials. b Analogues for animal leather. The MycoWorks technology is able to tune fine mycelium leather to have material advantages similar to animal skin, becoming supple, elastic and strong, with excellent return, drape, compression and insulation. This mycelium leather, launched in early 2020 as Reishi™, has been designed as a drop-in material for existing leather processing machine tools, where it can be cured, finished and manufactured using well-honed industrial techniques and formulas
Notably, mushroom-based materials can absorb and dissipate a variety of energetic forces, ranging from sounds to seismic waves to ballistics [ 54 ]. They are naturally flame retardant, good thermal insulators and can be grown as flexible or rigid as one desires [ 50 , 55 ]. While incredibly strong and durable, these materials can readily be broken down into constituent minerals and dispersed easily back into the world. Materials grown through a process of fermentation and decomposition require far less energy, water and other resources than conventional manufacturing.
Filamentous fungi and a wood-based bio-economy
In order to shift away from a fossil-based economy and mitigate climate change triggered by the continuous industrial overproduction of CO 2 , many countries around the world, including those of the European Union, support the transformation to a renewable, bio-based and resource-efficient economy. Wood is considered to play a key role in the efforts towards this goal, due to the large potential of forests and wood products to sequester carbon [ 56 , 57 ]. The term “sustainable” originates from forestry, initially coined by a German mining administrator, Hans Carl von Carlowitz, more than 300 years ago, who oversaw the timber supply of silver mines and realised that regrowth did not match the consumption rates [ 58 ]. Although the concept of “sustainability” has substantially broadened over time, it became a guiding concept in forestry, and recent surveys are demonstrating that European forests are still showing positive net annual increments [ 59 ] and are, therefore, actively storing carbon—a prerequisite for any economy that strives to become net carbon-neutral.
Current wood utilisation includes three major streams: energy, wood products, and pulp and paper, with about 60% going towards materials and 40% into energy in Europe. In this way, about 20% of the total carbon in use is annually sequestered into long-life materials [ 60 ]. However, in order to keep carbon stored within materials for as long as possible, a circular economy will be beneficial, including both recycling and reuse of wood products in a cascading fashion [ 61 ] before a final utilisation for energy services. Since an increased demand for and scarcity of wood can be expected with the transition to a bio-based economy, such resource efficiency will become even more important in the future. While high-quality wood would, therefore, be optimally used as timber for solid products initially, later utilisation steps in a virtual cascade would focus on the inherent fibres, polymers and monomeric wood constituents, such as sugars and lignans. This is where filamentous fungi have their highest potential to assist due to their natural ability of selectively and efficiently degrading and/or modifying all of these major wood constituents.
Due to the natural ability of fungi as heterotrophic organisms to efficiently degrade lignocellulosic plant biomass and convert the constituent sugars into energy-rich molecules, filamentous fungi or fungal enzyme cocktails have a high potential to be employed in the upgrading of wood in biorefinery applications towards second-generation biofuels or chemicals [ 62 ]. Up to now, however, mostly straws are used as substrate for these processes (e.g. the Sunliquid ® process by Clariant), with only a few examples of wood conversion (e.g. bioethanol from Borregaard); wood being much more recalcitrant, due to generally higher lignin contents among other things [ 63 ]. Considering all of the above, an integration of biotechnological processes using fungal platforms into pulp and paper mills which are already performing (chemical-based) wood separation, such as Kraft pulping, can be envisioned as having substantial technological and economic advantages [ 64 ].
Such “wood-based biorefineries” already exist—mostly in Scandinavia and eastern Europe [ 65 ]. Nevertheless, most of these are far from being true multi-product factories. Optimally, biorefineries would integrate biomass conversion processes and equipment to produce bioenergy and a range of products, including pulp, bio-based chemicals (e.g. for food and pharma), biofuels, electricity and heat. Regarding efficiency, the ability to use multiple feedstocks, such as those originating from pulping processes (pulpwood, extracts from effluents, fractions of pulping liquors), by-products from sawmills (e.g. wood shavings), or recovered materials (e.g. recycled paper and wood/fibre waste), would be advantageous. Many of these substrates are unsuitable for chemical upgrading due to their low purities and would have to undergo costly cleaning steps. Filamentous fungi, on the other hand, have the potential to circumvent these problems, since many are surprisingly robust against inhibitors and can selectively transform desired target compounds out of complex mixtures, including lignin-derived molecules [ 66 ].
In summary, wood-based biorefineries can play a central role in the transition towards sustainable economies, with the ability to generate commercial resources with a smaller carbon footprint of resources and emissions than traditionally produced materials (such as concrete). However, which product systems are the most promising ones regarding sustainability and efficiency must be assessed. Filamentous fungi can be of great value in these efforts and help to move more wood from energy into material use and, thus, to higher value creations. Nevertheless, substantial challenges and knowledge gaps can be identified that need to be addressed to be successful in the mid- to long-term:
Research in wood decomposition is lagging behind that for more readily degradable biomass, such as agricultural residues and straws.
Considering the benefits of using recovered (pre-used) wood products, pulp mill side streams and forestry/wood industry residues, the research and adaptation of fungal cell factories should include these to a much greater extent than done today.
Climate change is slowly altering the tree composition, and it can be expected that hardwoods, such as beech and oak, will replace the more drought- and pathogen-sensitive softwoods, such as the currently predominating spruce, in the future. These types of wood differ in their composition, with clear implications for fungal degradation [ 67 , 68 ]. Directed or engineered fungal adaptation and the optimisation of fungal enzyme cocktails will be needed to address these differences in order to optimise utilisation.
Lignin is the most underutilised of all wood polymers due to its complex structure and difficulties in processing. It forms an amorphous network of phenyl propane units coupled to hemicellulose via ester linkages. It is the second most abundant natural material on Earth after cellulose. The worldwide production of lignin is approximately 100 million tons annually [ 69 ]. One of the main challenges of lignin valorisation lies in its diverse structure and poor solubility. Most of the lignin obtained (Kraft lignin/black liquor) is incinerated directly in the pulping plants to recover energy. Only about 2% of the technical lignin globally available (~ 5 million tons) is currently utilised. Basidiomycetes are unique in their vast abilities to modify and/or mineralise lignin, and these organisms should play a central role in developing the utility of this highly promising polyphenol. The potential of lignin as a material reservoir will be achieved through much more rigorous characterisation of the structure/function predictability achieved through these fungal transformations.
Filamentous fungi to mitigate plastic pollution
Plastics are widely used in the global economy because they are inexpensive, versatile, lightweight and durable. Millions of tons of plastics accumulate in the environment annually due to their stability, poor recycling and low circular use, and they represent an ecological threat to nature and human health. At least 350–400 million tons of plastics are being produced annually worldwide [ 70 ], while its production keeps increasing by an average of 7% per annum. In 2014, for example, 311 million tons were produced, among these, 59 million tons in Europe. About 64% of the total European plastic demand is concentrated in five countries: Germany, Italy, France, the United Kingdom and Spain. The main types of plastics are polyethylene, polypropylene, polyvinyl chloride and polystyrene [ 71 ]. In 2014, the plastic waste in Europe amounted to 25.8 million tons that were recycled (30%), subjected to incineration for energy recovery (40%) or sent to landfill (30%). Efficient recycling and incineration are only practiced in a few countries, while in most countries, more than 50% of plastic waste is landfilled [ 71 ]. The latter undergo photo oxidation, degradation and mechanical disruption that often give rise to small fragments, i.e. microplastic particles [ 72 ]. Microplastics, plasticizers and plastic additives as well as added monomers and oligomers can enter surface and/or ground water and accumulate in the environment exerting toxic effects [ 73 ]. Approximately 5 to 13 million tons of plastic waste enters the Earth’s oceans every year, as the intentional or unintentional final destination [ 74 ]. When this waste is ingested by marine animals, it accumulates in the food chain [ 72 ]. The most abundant type of primary microplastics in water environments are fibres [ 75 ] that originate from washing synthetic textiles. Replacing synthetic textiles with mycelium-based textiles can be a means to greatly reduce plastic pollution. In addition, fungal materials could replace plastics in a variety of other applications (see above).
Current plasticizers are used, among other things, to improve the workability of concrete, the construction material most used in the world. Plasticizers enable the use of less water by keeping the same viscosity of the concrete, thereby producing a highly workable material that hardens into a strong final product. Plasticizers are typically not covalently bound to the polymers, which, over time, may lead to environmental contamination via leaching [ 73 ]. A bio-based alternative to the current fossil-based concrete plasticisers would be lignin functionalized to a higher solubility by the action of fungal or bacterial laccases or other oxidative enzymes. The very hydrophobic lignin is insoluble in water and alcohol but soluble in alkaline solutions. Fungal laccases are prevalent in asco- and basidiomycetes and are typically most active at low pH values of 3–5.5, which is a drawback for their use in functionalizing lignin. Accordingly, laccases have to be identified or engineered that have a shifted optimum pH towards an alkaline pH. One recent successful approach at AB Enzymes has been the engineering of a laccase from the ascomycete Melanocarpus albomyces using a directed evolution approach, which has led to threefold improved kinetics at pH 9.8 [ 76 ]. Notably, the principle applicability of laccase-functionalized lignin has been shown, but immediate commercial implementation is hindered by the general availability and inexpensive cost of fossil-based plasticizers.
To date, very little is known about the biodegradability of petroleum-derived polymers and plastics with fungi and only a few reports are available in the literature. Many basidiomycetes are generally known for their ability to degrade polycyclic aromatic hydrocarbons and are applied in bioremediation processes of contaminated soils and liquids [ 77 , 78 , 79 , 80 ]. So far, plastic-degrading fungi have been successfully isolated from weathered plastic waste obtained from marine habitats and terrestrial waste treatment facilities. The main fungal genera that were isolated and used for lab-scale plastic degradation, primarily of PET and polyurethane so far are: Acremonium , Alternaria , Aspergillus , Aureobasidium , Cladosporium , Debaryomyces , Emericella , Fusarium , Gliocladium , Mucor , Nectria , Neonectria , Penicillium , Phoma, Plectosphaerella , Rhizopus and Trichoderma [ 81 , 82 , 83 , 84 ]. In addition, enzymes produced by basidiomycetes ( Agrocybe aegerita, Auricularia auricular - judae, Bjerkandera adusta, Nematoloma frowardii, Pycnoporus cinnabarinus, Stropharia rugosoannulata ) have been identified and used for plastic degradation. Among the most promising enzymes used for PET and polyurethane treatment have been esterases, cutinases, lipases and lignin-modifying and unspecific macromolecule-depolymerising and hydrolytic enzymes, such as different peroxidases, laccases, glucose oxidases and cytochromes P450. However, no distinct degradation effects for virgin and pre-treated plastics have been achieved solely by the use of pure enzymes [ 70 ]. Hence, currently ongoing research is focused on mycelial-based degradation (whole cell degradation) of pure or mixed cultures in combination with purified enzyme cocktails.
The Janus face of filamentous fungi
The fungal kingdom is huge: six million species are estimated to exist on Earth [ 85 ], of which only a small proportion is known. Most are saprophytes and feed on dead plants and animals, and only a few dozen are exploited in biotechnology as cell factories. Leveraging their metabolic capacities and flexibilities will be key to achieving the circular economy outlined above (Fig. 2 ).
However, many filamentous fungi pose a threat to plant, animal and human life. As comprehensively covered in the first white paper of the EUROFUNG consortium published in 2016 [ 4 ], a multitude of plant-pathogenic fungi destroy staple crops annually, which would be enough to feed 600 million people [ 86 ]. Human-pathogenic fungi compromise the life of 1.2 billion people worldwide and kill about 1.5–2 million annually, exceeding the fatalities caused by malaria or tuberculosis [ 87 ]. Regarding more information on the huge impact of fungal pathogens on human health and food security and the related challenges, the interested reader is referred to the EUROFUNGs white paper from 2016 [ 4 ], a review covering the top 10 most feared fungi published in 2018 [ 88 ] and to the One Health report published by the American Academy of Microbiology in 2019 [ 89 ].
Meeting the agricultural production required to feed the increasing world population [ 90 ] is likely to be made more difficult by climate change. This problem is exacerbated by a wide range of plant diseases caused by the diverse group of fungal and newly emerging pathogens. A list of the ten most important fungal pathogens of plants published in 2012 [ 91 ] is outdated. If this list had been collated more recently it would have undoubtedly also included the basidiomycete Phakopsora pachyrhizi , which causes Asian soybean rust and threatens the sustainability of the millions of hectares of soybean grown in Brazil each year, despite only being detected in Brazil for the first time in 2001 [ 92 ]. Soybean production is now only viable in Brazil because of the availability of effective fungal control agents. However, even with the solutions available from the agricultural industry, crop losses remain high in soybean and a wide range of other crops [ 93 ]. In many cases, the losses seen are due to fungi that have developed resistance to existing control strategies [ 94 , 95 , 96 ]. The speed with which many species of fungi evolve and become resistant to existing control strategies means that there is a constant need to identify agents with novel modes of action. However, the development of novel fungicides by companies active in this field, such as Bayer Crop Science and Syngenta, requires a huge effort and major investment (Fig. 7 ), taking more than 10 years from the identification of a new screening ‘hit’ to market [ 97 ].
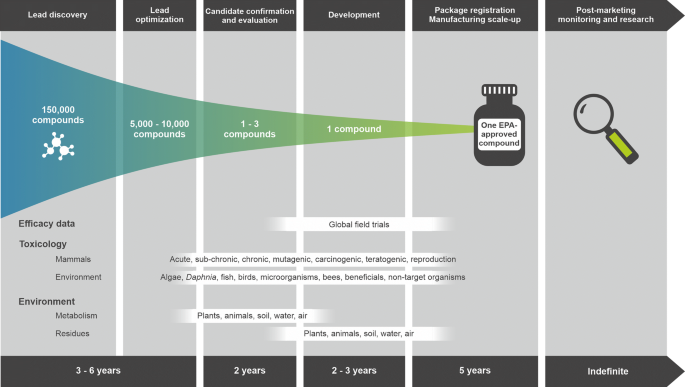
From science to market—the fungicide discovery and approval pipeline. Historically, the biological activity profile (fungicidal potency and spectrum) was used to filter hit compounds to lead molecules. Nowadays, regulatory requirements regarding human and ecotoxicological safety mean that proxy assays for these regulatory requirements are now utilised as early in the discovery pipeline as possible as part of the selection criteria for compound progression. It will typically take 10–12 years for a new fungicide to pass through the various stages of research (lead generation, early and late lead evaluation, optimisation and candidate confirmation) before promotion into evaluation and, finally, product development. After reaching the market, a significant amount of investment is still required for product lifecycle management (e.g. product monitoring feeds into improvements in formulation)
The screening of hundreds of thousands of random chemical inputs has historically produced a very low yield of hits, therefore, hypothesis-driven input selection methods are increasingly being utilised. However, the current target assessment and prioritization approaches are still heavily reliant on information from ‘model’ fungal systems, such as S. cerevisiae and Neurospora crassa , rather than on good quality data produced from fungal pathogens that result in the highest crop losses. It is remarkable that almost all plant pathogens diverged from the fungal ‘model’ systems millions of years ago and have very diverse modes of pathogenicity [ 98 , 99 , 100 ]. Therefore, the reliance on these classic ‘model’ systems is deeply unsatisfactory and a significantly better understanding of the genetics and metabolism of fungal plant pathogens is likely to help deliver novel fungal control strategies. In this respect, it is worth mentioning that the EUROFUNGs white paper from 2016 stressed that the term ‘model’ is misleading and advocated a shift in focus towards ‘reference’ strains [ 4 ].
Some ubiquitous filamentous fungi which are not pathogenic and usually thrive on dead plant material are still dangerous for human health when consuming crop-based products. Filamentous fungi produce a plethora of so-called secondary metabolites (SMs), of which a subset, the mycotoxins, are harmful. These can be produced by fungi growing either on the crops, some without disturbing plant growth, or post-harvest. The SMs are synthesised by accessory biosynthetic pathways thought to be important for a range of processes, including spore development, spore survival and fungal communication. Recent advances promote the view that fungal mycotoxins are fitness factors that help to establish a niche for protection from competing microbes [ 101 , 102 ].
Mycotoxins are toxic, carcinogenic and/or mutagenic fungal SMs and can be present in high concentrations in food and feed products. Mycotoxins have significant economic effects on a wide number of crops, most commonly but not exclusively contaminating grain crops (maize, wheat, barley), peanuts and tree nuts, beets, grapes and coffee. The Food and Agriculture Organization has estimated that 25% of the world’s crops are contaminated with unacceptable levels of mycotoxins. Economic losses are not easily quantified, but based on the reports that exist, losses are staggering. Aflatoxin, for example, is the most problematic mycotoxin, with estimated annual losses due to contamination ranging from $52 million to $1.68 billion regarding the US corn crop alone [ 103 ]. A relatively small number of ascomycete genera— Aspergillus, Penicillium, Fusarium, Claviceps and Alternaria —produce most of the common mycotoxins. Major mycotoxins include aflatoxins, citrinin, cyclopiazonic acid, ergot alkaloids, fumonisins, ochratoxin, patulin, trichothecenes and zearalenone [ 104 ]. All these metabolites have adverse effects on vertebrate cells depending on the amount ingested and the age and sex of the consumer. Aflatoxin B 1 is the mycotoxin most studied and the mechanism by which it causes liver cancer is well understood [ 105 ]. Trichothecenes, which interfere with protein synthesis in animals and humans, are common contaminates of wheat and a huge problem across all wheat-growing regions in the world. Patulin is of especial concern as it is frequently found in apples and products derived from apples that are popular children’s foods, such as apple sauce and juice [ 104 ]. It has also been proposed that SMs cause adverse human health effects after exposure by inhalation (e.g. exposure to moulds indoors; [ 106 ]).
Although the molecular genetics of many mycotoxins is well understood [ 104 ], efforts to control the contamination of food and feed with these small molecules is still to be realised. Aside from the challenges of controlling mycotoxin contamination using traditional efforts, such as breeding plants for resistance and/or detoxifying contaminated products, several emerging issues have complicated the ability to tackle the mycotoxin problem. Four significant issues to be considered in relation to mycotoxin amelioration are: (i) masked mycotoxins, (ii) climate change, (iii) emerging mycotoxins and (iv) co-occurrence of mycotoxins. Masked mycotoxins refer to plant derivatives of fungal mycotoxins. There is concern and data to support that these hybrid molecules can contribute to toxicity either directly or indirectly through the release of the parent mycotoxin in animal digestive tracks [ 107 ]. Research groups worldwide are now examining how climate change might impact mycotoxin synthesis for diverse fungi and crops. Several studies suggest increases in mycotoxin contamination although predictive modelling approaches need to be refined [ 108 , 109 , 110 ]. Emerging mycotoxins refers to toxic molecules that occur frequently in products but are not consistently monitored (e.g. eniatins, alternariol, emodin and sterigmatocystin; [ 111 ]). The frequency and occurrence of these less common mycotoxins should ideally be subject to risk assessment. Finally, many mycotoxins co-occur in the same crop, yet, worldwide regulations only consider the toxicology of a single mycotoxin [ 112 ]. Little is known whether combinations of mycotoxins affect the consumer in an antagonistic, additive or synergistic manner. Despite the wealth of published works on the topic of mycotoxins, substantial gaps remain in the knowledge of how to reduce contamination, what quantities are important in health, whether co-occurrence is a topic to consider and how climate change will impact future risks of these fungal metabolites.
Measures to advance science on filamentous fungi
Exploiting and fighting fungi for a sustainable, resource-saving bio-economy that operates in a circular manner demands considerable improvements in research and development on scientific and community levels:
Science: (i) improved understanding of fungal biology in a diverse set of species and strains, including lab (reference) strains, industrially exploited strains and ecologically relevant species; (ii) improved high-throughput technologies and tools to study the dynamics of fungal growth, product formation and pathogenicity and their adaptation to changing environmental conditions; (iii) investment in the standardisation of measures, methods and automation tools; and (iv) investment in open access infrastructures for the storage, analysis and reuse of systems biology data, including biofoundries.
Community: (i) overcoming fragmentation of the research community studying filamentous fungi; (ii) increasing the number of scientists devoting their lifetime to the study of fungi; (iii) the improved intersectoral and interdisciplinary education and training at undergraduate and graduate levels; and (iv) the engagement of scientists and educators in public outreach and the dissemination and engagement of the general public in fungal science.
The science on filamentous fungi has always been lagging behind that on many other microorganisms, such as yeast and bacteria. Four main reasons for this delay can be specified: firstly, genetic transformation of filamentous fungi is less efficient and more time-consuming compared to unicellular bacteria and yeast [ 113 ]. Secondly, genome sequences of filamentous fungi only became available a decade after the genomes of the ‘model’ unicellular fungus S. cerevisiae (1996; [ 114 ]) and the ‘model’ bacterium E. coli (1997; [ 115 ]) were released to the public. Thirdly, the genomes of filamentous fungi contain far more genes. A filamentous fungal genome usually carries between 9000 and 14,000 genes, whereas E. coli has about 4000 and S. cerevisiae about 6000 genes. Finally, there are a few million fungal species, consequently, the research communities studying a particular filamentous fungus are considerably small and fragmented. Each filamentous fungus is an individual of its own, different from all the other fungi, although its outlook is covered by the universal filamentous form. One of the largest of these scientific communities, studying A. niger , consists of only about 30 research labs worldwide [ 2 ], whereas more than 1800 research labs studying S. cerevisiae are registered at the Saccharomyces Genome Database [ 116 ].
Some substantial measures for basic and applied science on filamentous fungi are highlighted in the following. These need to be addressed in the short- to mid-term in order to fully leverage the power of these microorganisms for a circular economy.
Community engagement and sustainable infrastructures for high-quality fungal omics data
Biology has entered the digital age in many ways, most obviously with the rapid growth of large datasets, including genomes, transcriptomes, proteomes, phenomes and metabolomes. The exploitation of these data underpins the future of biology but requires effective infrastructure, supported and facilitated by easily accessible online technologies delivering high-value analyses. However, this revolution presents major technological challenges and resource implications. This is particularly stark for the fungal research community, due to the diversity of both the organisms and fields of research. On top of this, a vast amount of biological information and data is not in a searchable, digital form but is buried throughout our rich and varied literature.
Omics data is very cost-effective, but its utility is largely dependent on making the data accessible and usable for the wider research community. Every effort should be made to develop the future of genomics-driven fungal research in order that everyone can have full access to reliable genomic information. Consequently, accessing and representing diverse aspects of biology, ranging from phenotypes and subcellular localisation to protein interactions or the geographical location of isolates, in a searchable format is not easily achieved or readily automated. Therefore, a lot of the work required is time-consuming and requires dedicated personnel with biological expertise and information technology skills to apply appropriate classifications and ontologies. New solutions are needed for a large amount of multispecies omics data comparison. Addressing these issues requires co-ordination between researchers, publishers and databases, so that, at the time of publication, key information is deposited in a logical way within these resources. This will require the community to act proactively to facilitate the integration of data, consistency in the use of terminology and gene names, and journals to consider these issues when defining their requirements for data deposition and developing practices to facilitate text mining [ 117 ].
Fungal genome sequences are hosted at several fungal databases (e.g. FungiDB [ 118 ], JGI MycoCosm [ 119 ], Ensembl Fungi [ 120 ]), many more genomes are deposited in the NCBI, ENA or DDBJ, and some are hosted by private resources. Free, open access to data is generally accepted as a key priority, which, consequently, requires significant long-term funding for well maintained and resourced, actively developing databases to serve their user communities. Many funding organisations have contributed to setting up and maintaining bioinformatic resources, but the required long-term commitment is difficult to obtain, and with an inevitable cliff edge each time renewal of funding is sought, the reality is that key resources have been lost and will be lost in the future. A consequence of the funding issues is a general drive to centralise, with a number of organism-specific resources having been closed down or frozen (e.g. AspGD), due to a loss of funding. How to address this global issue is not easy and needs both engagement and commitment from funding agencies and/or governments, alongside concerted efforts from the community to shape policy. Alternative funding models must also be considered. However, most proposals potentially endanger the ethos of full open access.
Early genome sequences were limited in quality due mainly to the sequencing technologies used; more recent higher quality and better assembled genome sequences suffer from poor annotation. In the early days, consortia were formed to improve upon the automated gene calling and annotation by manual curation and verification of genes. Nowadays, very few genomes make it past the initial draft annotation [ 4 ]. Consequently, errors are likely to occur, and these will be perpetuated and propagated in the annotation of other genomes, influencing downstream experimental analysis. Additionally, comparison of outputs from different bioinformatic pipelines using the same datasets will generally not give exactly the same interpretation—the outputs represent probabilities and models are, thus, not absolute. The resulting differences inevitably complicate the comparison of genomes and other types of data. Lack of awareness or technical understanding can also lead to the misinterpretation and misinformation of any programme or bioinformatic output. This underlines the need for improved training and development, not only for dedicated bioinformaticians but even more for biologists in general. Currently, there is a serious lack of qualified personnel with adequate formal training. Consequently, as a minimum, there is a need for appropriate training regarding accepted rules for omics data handling, enabling fungal biologists to efficiently contribute their domain-specific expertise using ontologies, terminologies and platforms that minimise the need for professional curation and maximise value to the broader user community.
Databases, such as FungiDB and MycoCosm, have a challenge in choosing the organisms and datasets they should host and on where the particular reference genomes, their limited annotation and curation efforts should focus. Consequently, the active engagement with and involvement of researchers is essential in making these decisions. Most researchers inevitably regard their own work as a priority and there is a danger that those with the ‘sharpest elbows’ will prevail. Therefore, it is important that communities ensure they are well represented and informed of decisions, proposed priorities and opportunities.
Opportunities and challenges in getting value from fungal omics data
The integration of databases provides opportunities for shared resources and infrastructures which have inevitable cost-savings. ClinEpiDB [ 121 , 122 ], for example, accommodates a diverse range of large-scale epidemiological datasets for enteric, respiratory and parasitic pathogens but no fungal data. The common infrastructure used by ClinEpiDB and FungiDB could potentially facilitate the integration of epidemiological datasets for fungal pathogens and, as such, allow room for innovation. These resources, allowing the mapping of disease and genomic data, could be potentially developed more broadly for fungal researchers interested in plant disease, ecology and the migration of key pathogens or drug resistance. Additionally, this may facilitate new developments to include the characterisation of transcriptional changes associated with host pathogen interactions [ 123 ] or between microbiomes [ 124 ].
A major challenge for both the database providers and their users is that data is not static nor is the interpretation of data generally limited to a single definitive result. Genomes, for example, may be re-sequenced using new technologies and additional datasets, such as proteomics or RNA-Seq, are constantly being added. These changes and additions, alongside modifications to bioinformatic pipelines, will facilitate improved gene calling and annotation. Consequently, gene models may be removed or split, misannotated open reading frames revised, differential splicing revealed and untranslated regions, defining the transcription start sites and mature 3′ ends, clarified. These changes can result in difficulties. It is challenging, for example, to map all N. crassa knockout mutants to the current gene models hosted by different databases. Researchers, therefore, need to be aware that genomes are not set in stone but evolve with data and technical advances and the databases must make these changes clear and traceable. Updating repository records is paramount to ensure that all databases have access to the most recent information and, as such, provide the best possible service to their users.
Another confounding factor when harnessing genome data of filamentous fungi is the fact that between 40 and 50% of the genes in a filamentous fungal genome are ‘hypothetical’ and lack functional predictions [ 4 ]. In addition, only 2–10% of the genes with predicted functions have been experimentally studied in filamentous fungi and, thus, have a verified function [ 125 , 126 ]. This renders in silico reconstructed genome-wide metabolic models considerably incomplete, i.e. with gaps, dead-end reactions and dead-end metabolites. Even for the ‘model’ yeast S. cerevisiae , 21% of its predicted genes (i.e. about 1400 genes) still have dubious functional predictions in 2019, which is 22 years after the release of its genome sequence and despite a research community with more 1800 labs worldwide [ 127 ]. One powerful approach to overcome this limitation is the interrogation of gene expression networks based on hundreds of transcriptomic datasets available for filamentous fungi. This wealth of data has recently been harnessed to improve gene annotations and assign gene function predictions to 65% of genes predicted in the A. niger genome [ 128 ], which now can be accessed at FungiDB.
Notably, ecologically important pathogens and endophytic fungi have traditionally received very little support due to the absence of dedicated funding. Those managing the bioinformatics resource need to work with the researchers to define the types of data and criteria that apply when selecting datasets for inclusion so that the process is rigorous, not biased or arbitrary, and that appropriate computational workflows are developed in a timely way. This will also help the researchers to consider relevant criteria when designing their experiments and specific initiatives may be developed to address particular problems. A key issue in confirming gene models, for example, is identification of the 5’ and 3’ ends of transcripts. Most RNA-Seq approaches are poor at defining transcript ends, but specific approaches can be applied that address this directly. In this way, the structural annotation of key genomes could be significantly improved for the wider community. Awareness of such issues may help the research community optimise the utility of their data.
Other on-line resources provide bioinformatic pipelines to assist and minimise the expertise required. One example is Companion [ 129 ], which provides a genome production pipeline. The most recent version has proved effective in the production of usable fungal genomes based on the de novo analysis of 25 previously characterised reference genomes. The future development and continued support of this and other such initiatives should help the lab-based researchers complete a good first draft of a genome annotation efficiently without major resource implications. Furthermore, recent developments in the Apollo pipeline [ 130 ], integrated within databases such as VEuPathDB [ 131 ], allows researchers to inspect and modify genome features efficiently and can also be utilised in private workspaces. Data can remain private to a specific work group and be transferred to public databases when appropriate. Consequently, the research community can fully engage to develop shared resources. As these contributions can be attributed to their author, this can be acknowledged by the community and potentially lead to micropublications, incentivising participation and rewarding those who contribute.
Last but not least, the rapid evolution of sequencing and other high-throughput technologies requires active discussions to improve policies and infrastructure for data sharing, integration and analysis in order to resolve the bottlenecks and challenges faced. The changes will not happen overnight, but effective communication should involve all parties concerned, including the on-line database providers, researchers, funding agencies and policymakers. In order for these amazing resources to be used to their full potential for high impact research, these different parties must actively strive to ensure we have effective data sharing policies that will ultimately result in long-term sustainability, accessibility and utility.
Improved tools and technologies to study fungal biology
The molecular and analytical toolkit available for filamentous fungi has improved substantially during the 4 years since the first white paper was published in 2016 [ 4 ], demonstrating the high innovation potential of the filamentous fungal research community. Genome editing via CRISPR has become routine and is nowadays run in a multiplex manner for many reference strains [ 132 , 133 ]. Fourier-transformed infrared spectroscopy has become a next-generation phenotyping technology to identify fungal strains and their metabolic products [ 134 ], while the power of surface analysis tools, such as mass spectrometry-based imaging techniques, has been exploited to identify changes that are brought about by filamentous fungi and their enzymes when they attack and modify insoluble lignocellulose materials [ 135 ]. “Ready-to-use-microfluidics” have been implemented to study the dynamics of fungal growth and fungal interaction with soil bacteria [ 136 ], X-ray microcomputed tomography has been successfully applied to study spatial distribution of hyphae within mycelia and diffuse mass transport therein [ 137 , 138 ], and gene and protein networks have been developed to assist our understanding of filamentous fungal biology holistically [ 128 , 139 ].
Hence, the technological foundation is sound and powerful and no longer represents a critical hurdle for gaining knowledge on fungal biology. As of now, high-throughput methods for automated cloning, cultivation, mining and screening of fungal strains are, unfortunately, not yet state-of-the-art.
Standardisation as a driver for engineering fungal biology
Standardisation is a key for success. Standard reference methods, measures, substrates and materials ensure reliable fabrication and reproducible production characteristic of advanced global industries, such as the semiconductor and forest products industries [ 140 , 141 , 142 ]. Engineering biology, broadly called synthetic biology, is similarly benefitting from standardisation [ 143 , 144 ]. The mycelium materials research community and industry are already benefitting from ASTM and ISO standards developed for products in the forest, textile and acoustic absorbance industries. Accelerating the development and adoption of standard reference materials, measures and methods across the mycelial materials community and beyond could enable advancements in basic science on filamentous fungi and engineering of fungal-based bioproduction analogous to the advancements in the semiconductor industry. New options for sharing mycelium materials that reduce transaction costs, allow for redistribution and enable commercial production could provide the incentive for the broad adoption of standard references, measures and methods that support reliable fabrication and reproducible science [ 145 , 146 ].
Reliable, standardised measurements are critical to reproducible science [ 147 ]. Metrology formalizes reliable measurements, and advances in metrology for biological systems are greatly benefitting basic science and the engineering of biology [ 148 , 149 ]. Characterising organisms, substrates and production environments in real-time across orders of magnitude in the scale of space and time could accelerate the development of advanced fungal products while, simultaneously, benefitting basic science and the engineering of biological systems. As an example, standard metrological materials, such as well-characterised 1 cm 3 units of mycelium material, and metrological methods could help to co-ordinate practitioners across locations and resource settings, reduce manufacturing costs, reduce time to market and disseminate best practices [ 150 ].
The confluence of standard approaches and automation tools characteristic of modern semiconductor foundries have cultivated the semiconductor industry into one of the most significant in human history. Recent advancements in the automated construction of synthetic organisms are making biofoundries economically viable and they are increasingly being recognized by the life sciences research community as an essential infrastructure for basic and applied research [ 151 , 152 ]. Companies such as Gingko Bioworks and Ecovative Design have invested substantial capital in biofoundries for constructing organisms and there is an emerging ecosystem of “cloud laboratories” that aim to provide practitioners access to automated labs without the need for large capital investments [ 153 , 154 , 155 , 156 ]. Biofoundries with capacities for constructing filamentous fungal organisms could build on recent work to synthesize the genomes of the bacteria E. coli, Caulobacter crescentus and the yeast S. cerevisiae , with the ultimate goal of enabling advancements in synthetic filamentous fungal organism construction analogous to the construction of JCVI-sc3.0 and Sc2.0 [ 157 , 158 , 159 , 160 ]. Work to insulate biological systems from evolutionary drift can enable the deployment of engineered biology for applications outside the research laboratory or commercial bioreactors, while mitigating biosafety and biosecurity risks [ 161 ]. Such is the promise of distributed fungal-based bioproduction that biofoundries, for example, for mycelium materials in support of basic science, engineering and production, strategically placed across the planet, could ramify into impacts surpassing that of the semiconductor industry.
Concluding remarks
We would not be able to live the life we are living without the help of moulds and mushrooms from nature. Fungi are our present and they will shape our future. They are champions in recycling and material transformation; their biosynthetic capacities are unmatched in the microbial world. We should do our best to harness their abilities! Fundamental and applied science on fungi offers solutions for the shift from our current petroleum-based economy into a bio-based circular economy, opens new avenues for food security as demand increases from a growing human population, and provides us with new concepts on how to ensure human, animal and plant health in the future. Science on fungi discussed in this white paper already contributes to 10 out of 17 UN development goals (Fig. 8 ) and their role will become even more important for the future of mankind.
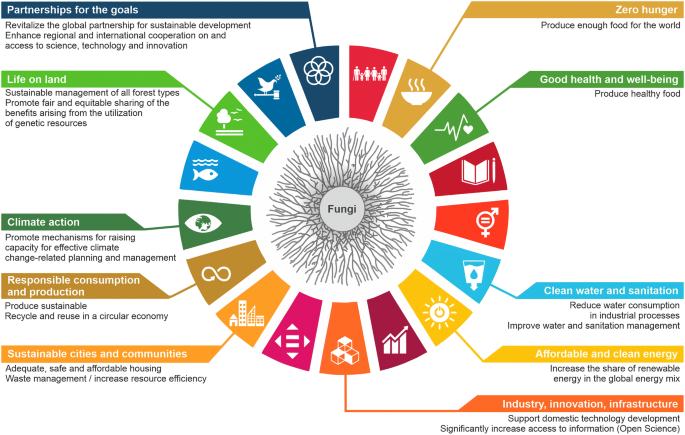
Fungal biotechnology has the potential to make a significant contribution meeting 10 out of 17 United Nation’s sustainable development goals through the rational improvement of filamentous fungal cell factories
The economist Peter Drucker (1909–2005) once said: “The best way to predict your future is to create it.” Stronger mutual collaborations between scientists, engineers, artists, designers and industrial stakeholders, and vivid communication with the general public and policymakers will ensure that the inter- and transdisciplinary science on fungi will create a path towards innovative breakthroughs. As Peter Drucker suggests, this will create a sustainable economic future based on fungal cell factories for years to come.
Availability of data and materials
Not applicable.
Ereky K. Biotechnologie der Fleisch-, Fett-, und Milcherzeugung im landwirtschaftlichen Grossbetriebe: für naturwissenschaftlich gebildete Landwirte verfasst. Berlin: Paul Parey; 1919.
Google Scholar
Cairns TC, Nai C, Meyer V. How a fungus shapes biotechnology: 100 years of Aspergillus niger research. Fungal Biol Biotechnol. 2018;5:13.
Article PubMed PubMed Central Google Scholar
Citric Acid Market worth 3.6 Billion USD by 2020. Markets and markets. 2015. https://www.marketsandmarkets.com/PressReleases/citric-acid.asp . Accessed 3 Jan 2020.
Meyer V, Andersen MR, Brakhage AA, Braus GH, Caddick MX, Cairns TC, et al. Current challenges of research on filamentous fungi in relation to human welfare and a sustainable bio-economy: a white paper. Fungal Biol Biotechnol. 2016;3:6.
Bayer E. The mycelium revolution is upon us. Scientific American. 1 July 2019. https://blogs.scientificamerican.com/observations/the-mycelium-revolution-is-upon-us/ . Accessed 20 Feb 2020.
Hyde KD, Xu J, Rapior S, Jeewon R, Lumyong S, Niego AGT, et al. The amazing potential of fungi: 50 ways we can exploit fungi industrially. Fungal Divers. 2019;97:1–136. https://doi.org/10.1007/s13225-019-00430-9 .
Article Google Scholar
Smith ML, Bruhn JN, Anderson JB. The fungus Armillaria bulbosa is among the largest and oldest living organisms. Nature. 1992;356:428–31.
Pelkmans JF, Patil MB, Gehrmann T, Reinders MJ, Wösten HA, Lugones LG. Transcription factors of Schizophyllum commune involved in mushroom formation and modulation of vegetative growth. Sci Rep. 2017;7:310.
Article PubMed PubMed Central CAS Google Scholar
Spatafora JW, Aime MC, Grigoriev IV, Martin F, Stajich JE, Blackwell M. The fungal tree of life: from molecular systematics to genome-scale phylogenies. Microbiol Spectr. 2017. https://doi.org/10.1128/microbiolspec.funk-0053-2016 .
Article PubMed Google Scholar
Bar-On YM, Phillips R, Milo R. The biomass distribution on Earth. Proc Natl Acad Sci USA. 2018;115:6506–11.
Article CAS PubMed PubMed Central Google Scholar
Cherry JR, Fidantsef AL. Directed evolution of industrial enzymes: an update. Curr Opin Biotechnol. 2003;14:438–43.
Article CAS PubMed Google Scholar
van den Brink J, de Vries RP. Fungal enzyme sets for plant polysaccharide degradation. Appl Microbiol Biotechnol. 2011;91:1477–92.
Meyer V. Metabolic engineering of filamentous fungi. In: Lee, Nilesen, Stephanopoulos, editors. Metabolic engineering. Concepts and applications. Wiley, in press; 2020.
van den Berg MA, Albang R, Albermann K, Badger JH, Daran JM, Driessen AJ, et al. Genome sequencing and analysis of the filamentous fungus Penicillium chrysogenum . Nat Biotechnol. 2008;26:1161–8. https://doi.org/10.1038/nbt.1498 .
Houbraken J, Frisvad JC, Samson RA. Fleming’s penicillin producing strain is not Penicillium chrysogenum but P. rubens . IMA Fungus. 2011;2:87–95.
Business Wire. FDA grants breakthrough therapy designation to Usona Institute’s psilocybin program for major depressive disorder. 22 November 2019. https://www.businesswire.com/news/home/20191122005452/en/FDA-grants-Breakthrough-Therapy-Designation-Usona-Institutes . Accessed 20 Feb 2020.
Karaffa L, Kubicek CP. Citric acid and itaconic acid accumulation: variations of the same story? Appl Microbiol Biotechnol. 2019;103:2889–902.
Kuivanen J, Wang YJ, Richard P. Engineering Aspergillus niger for galactaric acid production: elimination of galactaric acid catabolism by using RNA sequencing and CRISPR/Cas9. Microb Cell Fact. 2016;15:210.
Dai Z, Zhou H, Zhang S, Gu H, Yang Q, Zhang W, et al. Current advance in biological production of malic acid using wild type and metabolic engineered strains. Bioresour Technol. 2018;258:345–53.
Liu J, Li J, Shin HD, Du G, Chen J, Liu L. Biological production of L-malate: recent advances and future prospects. World J Microbiol Biotechnol. 2017;34:6.
Article PubMed CAS Google Scholar
Teleky BE, Vodnar DC. Biomass-derived production of itaconic acid as a building block in specialty polymers. Polymers (Basel). 2019;11:E1035.
Junod SW. Statins: a success story involving FDA, academia and industry. Food and Drug Administration. The article originally appeared in the “History Corner” column of the March–April 2007 issue of Update magazine. https://www.fda.gov/media/110452/download . Accessed 25 Sept 2019.
Barrios-González J, Miranda RU. Biotechnological production and applications of statins. Appl Microbiol Biotechnol. 2010;85:869–83.
Liang B, Huang X, Teng Y, Liang Y, Yang Y, Zheng L, et al. Enhanced single-step bioproduction of the simvastatin precursor monacolin J in an industrial strain of Aspergillus terreus by employing the evolved lovastatin hydrolase. Biotechnol J. 2018;13:e1800094.
Huang X, Tang S, Zheng L, Teng Y, Yang Y, Zhu J, et al. Construction of an efficient and robust Aspergillus terreus cell factory for monacolin J production. ACS Synth Biol. 2019;8:818–25.
van den Berg MA. Impact of the Penicillium chrysogenum genome on industrial production of metabolites. Appl Microbiol Biotechnol. 2011;92:45–53.
McLean KJ, Hans M, Meijrink B, van Scheppingen WB, Vollebregt A, Tee KL, et al. Single-step fermentative production of the cholesterol-lowering drug pravastatin via reprogramming of Penicillium chrysogenum . Proc Natl Acad Sci USA. 2015;112:2847–52.
Blumer-Schuette SE, Brown SD, Sander KB, Bayer EA, Kataeva I, Zurawski JV, et al. Thermophilic lignocellulose deconstruction. FEMS Microbiol Rev. 2014;38:393–448.
Burdette LA, Leach SA, Wong HT, Tullman-Ercek D. Developing Gram-negative bacteria for the secretion of heterologous proteins. Microb Cell Fact. 2018;17:196.
Hou J, Tyo KE, Liu Z, Petranovic D, Nielsen J. Metabolic engineering of recombinant protein secretion by Saccharomyces cerevisiae . FEMS Yeast Res. 2012;12:491–510.
Zhou Y, Raju R, Alves C, Gilbert A. Debottlenecking protein secretion and reducing protein aggregation in the cellular host. Curr Opin Biotechnol. 2018;53:151–7.
Ilica RA, Kloetzer L, Galaction AI, Caşcaval D. Fumaric acid: production and separation. Biotechnol Lett. 2019;41:47–57.
Li J, Lin L, Sun T, Xu J, Ji J, Liu Q, et al. Direct production of commodity chemicals from lignocellulose using Myceliophthora thermophila . Metab Eng. 2019. https://doi.org/10.1016/j.ymben.2019.05.007 .
Dyadic International Inc. C1 expression system. https://www.dyadic.com/c1-technology/c1-expression-system/ . Accessed 3 Jan 2020.
Moore D, Chiu SW. Fungal products as food. In: Pointing SB, Hyde KD, editors. Bio-exploitation of filamentous fungi., Fungal Diversity Research Series 6London: Fungal Diversity Press; 2001. p. 223–51.
Mycorena. Creating green protein with no plants. https://mycorena.com/ . Accessed 20 Feb 2020.
Sustainable Bioproducts. https://www.sustainablebioproducts.com/ . Accessed 20 Feb 2020.
MycoTechnology. How mushrooms are transforming the food industry. http://redesign.mycotechcorp.com/ . Accessed 20 Feb 2020.
Finnigan TJA, Wall BT, Wilde PJ, Stephens FB, Taylor SL, Freedman MR. Mycoprotein: the future of nutritious nonmeat protein, a symposium review. Curr Dev Nutr. 2019;3:nzz021. https://doi.org/10.1093/cdn/nzz021 .
Carbon Trust. Quorn—product carbon footprinting and labelling. https://www.carbontrust.com/our-clients/q/quorn-product-carbon-footprinting-and-labeling/ . Accessed 20 Feb 2020.
Merzendorfer H. The cellular basis of chitin synthesis in fungi and insects: common principles and differences. Eur J Cell Biol. 2011;90:759–69.
Grimm D, Wösten HAB. Mushroom cultivation in the circular economy. Appl Microbiol Biotechnol. 2018;102:7795–803.
Islam MR, Tudryn G, Bucinell R, Schadler L, Picu RC. Mechanical behavior of mycelium-based particulate composites. J Mater Sci. 2018;53:16371–82.
Article CAS Google Scholar
Pelletier MG, Holt GA, Wanjura JD, Lara AJ, Tapia-Carillo A, McIntyre G, et al. An evaluation study of pressure-compressed acoustic absorbers grown on agricultural by-products. Ind Crops Prod. 2013;95:342–7.
Appels FVW, Camere S, Montalti M, Karana E, Jansen KMB, Dijksterhuis J, et al. Fabrication factors influencing mechanical, moisture- and water-related properties of mycelium-based composites. Mater Des. 2019;161:64–71.
Attias N, Danai O, Abitbol T, Tarazi E, Ezov N, Pereman I, et al. Mycelium bio-composites in industrial design and architecture: comparative review and experimental design. J Clean Prod. 2020;246:119037.
Appels FVW, Dijksterhuis J, Lukasiewicz CE, Jansen KMB, Wösten HAB, Krijgsheld P. Hydrophobin gene deletion and environmental growth conditions impact mechanical properties of mycelium by affecting the density of the material. Sci. Rep. 2018;8:4703.
Nai C, Meyer V. The beauty and the morbid: fungi as source of inspiration in contemporary art. Fungal Biol Biotechnol. 2016;3:10.
Meyer V. Merging science and art through fungi. Fungal Biol Biotechnol. 2019;6:5.
Mycoworks. Reishi. https://www.mycoworks.com/ . Accessed 20 Feb 2020.
Ecovative Design. https://ecovativedesign.com/ . Accessed 20 Feb 2020.
Neffa. https://neffa.nl/ . Accessed 20 Feb 2020.
Mogu. https://mogu.bio/ . Accessed 20 Feb 2020.
Pelletier MG, Holt GA, Wanjura JD, Grettham L, McIntyre G, Bayer E, et al. Acoustic evaluation of mycological biopolymer, an all-natural closed cell foam alternative. Ind Crops Prod. 2019;139:111533.
Jones M, Bhat T, Kandare E, Thomas A, Joseph P, Dekiwadia C, et al. Thermal degradation and fire properties of fungal mycelium and mycelium—biomass composite materials. Sci Rep. 2018;8:17583. https://doi.org/10.1038/s41598-018-36032-9 .
WBAE/WBW: Scientific Advisory Board on Agricultural Policy, Food and Consumer Health Protection (WBAE) and on Forest Policy (WBW) at the Federal Ministry of Food and Agriculture (BMEL). In: (2016) Climate change mitigation in agriculture and forestry and in the downstream sectors of food and timber use. Executive Summary. Berlin; 2016. https://doi.org/10.12767/buel.v1i1.175.g323 .
Nabuurs GJ, Verkerk PJ, Schelhaas MJ, Olabarria JRG, Trasobares A, Cienciala E. Climate-smart forestry: mitigation impacts in three European regions. From Science to Policy 6. European Forest Institute; 2018.
Von Carlowitz HC. Sylvicultura oeconomica oder Haußwirthliche Nachricht und Naturmäßige Anweisung zur Wilden Baum-Zucht. Hamberger J, editor. Oekom verlag; 2013 [1713].
Forest Europe. State of Europe’s Forests 2015. Ministerial Conference on the Protection of Forests in Europe. Forest Europe Liaison Unit Madrid. 2015. https://foresteurope.org/state-europes-forests-2015-report/ . Accessed 21 Feb 2020.
EASAC—European Academies’ Science Advisory Council. Multi-functionality and sustainability in the European Union’s forests. EASAC policy report 32. 2017. https://easac.eu/publications/details/multi-functionality-and-sustainability-in-the-european-unions-forests/ . Accessed 21 Feb 2020.
Höglmeier K, Weber-Blaschke G, Richter K. Potentials for cascading of recovered wood from building deconstruction—a case study for south-east Germany. Resour Conserv Recy. 2017;117:304–14.
Lange L. Fungal enzymes and yeasts for conversion of plant biomass to bioenergy and high-value products. Microbiol Spectr. 2017. https://doi.org/10.1128/microbiolspec.funk-0007-2016 .
Ragauskas AJ, Beckham GT, Biddy MJ, Chandra R, Chen F, Davis MF, et al. Lignin valorization: improving lignin processing in the biorefinery. Science. 2014;344:1246843. https://doi.org/10.1126/science.1246843 .
Hetemäki L, editor. Future of the European forest-based sector: structural changes towards bioeconomy. European Forest Institute: Joensuu; 2014.
Bio-based Industries Consortium & nova-Institut GmbH. Biorefineries in Europe 2017. https://biconsortium.eu/downloads/biorefineries-europe-2017 . Accessed 21 Feb 2020.
Yaegashi J, Kirby J, Ito M, Sun J, Dutta T, Mirsiaghi M, et al. Rhodosporidium toruloides : a new platform organism for conversion of lignocellulose into terpene biofuels and bioproducts. Biotechnol Biofuels. 2017;10:241.
Hassan L, Reppke MJ, Thieme N, Schweizer SA, Mueller CW, Benz JP. Comparing the physiochemical parameters of three celluloses reveals new insights into substrate suitability for fungal enzyme production. Fungal Biol Biotechnol. 2017;4:10.
Novy V, Nielsen F, Seiboth B, Nidetzky B. The influence of feedstock characteristics on enzyme production in Trichoderma reesei : a review on productivity, gene regulation and secretion profiles. Biotechnol Biofuels. 2019;12:238.
Bajwa DS, Pourhashem G, Ullah AH, Bajwa SG. A concise review of current lignin production, applications, products and their environmental impact. Ind Crops Prod. 2019;139:111526.
Danso D, Chow J, Streit WR. Plastics: environmental and biotechnological perspectives on microbial degradation. Appl Environ Microbiol. 2019;85:e01095-19.
Plastics Europe. Association of plastics manufacturers. https://www.plasticseurope.org/ . Accessed 21 Feb 2020.
Teuten EL, Saquing JM, Knappe DR, Barlaz MA, Jonsson S, Björn A, et al. Transport and release of chemicals from plastics to the environment and to wildlife. Philos Trans R Soc Lond B Biol Sci. 2009;364:2027–45. https://doi.org/10.1098/rstb.2008.0284 .
Gregory MR. Environmental implications of plastic debris in marine settings—entanglement, ingestion, smothering, hangers-on, hitch-hiking and alien invasions. Philos Trans R Soc Lond B Biol Sci. 2009;364:2013–25. https://doi.org/10.1098/rstb.2008.0265 .
Jambeck JR, Geyer R, Wilcox C, Siegler TR, Perryman M, Andrady A, et al. Marine pollution. Plastic waste inputs from land into the ocean. Science. 2015;347:768–71. https://doi.org/10.1126/science.126035 .
Boucher J, Friot D. Primary microplastics in the oceans. A global evaluation of sources. IUCN. 2017. https://doi.org/10.2305/iucn.ch.2017.01.en .
Novoa C, Dhoke GV, Mate DM, Martinez R, Haarmann T, Schreiter M, et al. KnowVolution of a fungal laccase toward alkaline pH. ChemBioChem. 2019;20:1458–66.
Asgher M, Bhatti HN, Ashraf M, Legge RL. Recent developments in biodegradation of industrial pollutants by white rot fungi and their enzyme system. Biodegradation. 2008;19:771–83. https://doi.org/10.1007/s10532-008-9185-3 .
Patent: CN103013841A Scopulariopsis brevicaulis and application thereof. https://patents.google.com/patent/CN103013841A/en . Accessed 21 Feb 2020.
Mao J, Guan W. Fungal degradation of polycyclic aromatic hydrocarbons (PAHs) by Scopulariopsis brevicaulis and its application in bioremediation of PAH-contaminated soil. Acta Agr Scand Sec B. 2016;66:399–405. https://doi.org/10.1080/09064710.2015.1137629 .
Ezekoye CC, Chikere CB, Okpokwasili GC. Fungal diversity associated with crude oil-impacted soil undergoing in-situ bioremediation. Sustain Chem Pharm. 2018;10:148–52. https://doi.org/10.1016/j.scp.2018.11.003 .
Pathak VM. Navneet Review on the current status of polymer degradation: a microbial approach. Bioresour Bioprocess. 2017;4:15. https://doi.org/10.1186/s40643-017-0145-9 .
Bentham RH, Morton LHG, Allen NG. Rapid assessment of the microbial deterioration of polyurethanes. Int Biodeterior. 1987;23:377–86. https://doi.org/10.1016/0265-3036(87)90026-1 .
Ghosh SK, Pal S, Ray S. Study of microbes having potentiality for biodegradation of plastics. Environ Sci Pollut Res Int. 2013;20:4339–55. https://doi.org/10.1007/s11356-013-1706-x .
Russell JR, Huang J, Anand P, Kucera K, Sandoval AG, Dantzler KW, et al. Biodegradation of polyester polyurethane by endophytic fungi. Appl Environ Microbiol. 2011;77:6076–84. https://doi.org/10.1128/AEM.00521-11 .
Taylor DL, Hollingsworth TN, McFarland JW, Lennon NJ, Nusbaum C, Ruess RW. A first comprehensive census of fungi in soil reveals both hyperdiversity and fine-scale niche partitioning. Ecol Monogr. 2014;84:3–20. https://doi.org/10.1890/12-1693.1 .
Fisher MC, Hawkins NJ, Sanglard D, Gurr SJ. Worldwide emergence of resistance to antifungal drugs challenges human health and food security. Science. 2018;360:739–42. https://doi.org/10.1126/science.aap7999 .
Denning DW, Bromley MJ. Infectious disease. How to bolster the antifungal pipeline. Science. 2015;347:1414–6. https://doi.org/10.1126/science.aaa6097 .
Hyde KD, Al-Hatmi AMS, Andersen B, Boekhout T, Buzina W, Dawson TJ Jr, et al. The world’s ten most feared fungi. Fungal Divers. 2018;93:161–94. https://doi.org/10.1007/s13225-018-0413-9 .
Konopka JB, Casadevall A, Taylor JW, Heitman J, Cowen L. One health: fungal pathogens of humans, animals, and plants. Report on an American Academy of Microbiology Colloquium held in Washington, DC, on 18 October 2017. Washington (DC): American Society for Microbiology; 2019.
How to feed the world by 2050. FAO. http://www.fao.org/fileadmin/templates/wsfs/docs/expert_paper/How_to_Feed_the_World_in_2050.pdf . Accessed 21 Feb 2020.
Dean R, Van Kan JA, Pretorius ZA, Hammond-Kosack KE, Di Pietro A, Spanu PD, et al. The Top 10 fungal pathogens in molecular plant pathology. Mol Plant Pathol. 2012;13:414-30. https://doi.org/10.1111/j.1364-3703.2011.00783.x . Review. Erratum in: Mol Plant Pathol. 2012;13:804.
Yorinori JT, Paiva WM, Frederick RD, Costamilan JM, Vertagnolli PF, Hartman GE, et al. Epidemics of soybean rust ( Phakopsora pachyrhizi ) in Brazil and Paraguay from 2001 to 2003. Plant Dis. 2005;89:675–7. https://doi.org/10.1094/PD-89-0675 .
Savary S, Willocquet L, Pethybridge SJ, Esker P, McRoberts N, Nelson A. The global burden of pathogens and pests on major food crops. Nat Ecol Evol. 2019;3:430–9. https://doi.org/10.1038/s41559-018-0793-y .
Pretorius ZA, Singh RP, Wagoire WW, Payne TS. Detection of virulence to wheat stem rust resistance gene Sr31 in Puccinia graminis . f. sp. tritici in Uganda. Plant Dis. 2000;84:203. https://doi.org/10.1094/pdis.2000.84.2.203b .
Fernández-Ortuño D, Pérez-García A, Chamorro M, de la Peña E, de Vicente A, Torés JA. Resistance to the SDHI fungicides boscalid, fluopyram, fluxapyroxad, and penthiopyrad in Botrytis cinerea from commercial strawberry fields in Spain. Plant Dis. 2017;101(7):1306–13.
Steinhauer D, Salat M, Frey R, Mosbach A, Luksch T, Balmer D, et al. A dispensable paralog of succinate dehydrogenase subunit C mediates standing resistance towards a subclass of SDHI fungicides in Zymoseptoria tritici . PLoS Pathog. 2019;15:e1007780. https://doi.org/10.1371/journal.ppat.1007780 .
Nishimoto R. Global trends in the crop protection industry. J Pestic Sci. 2019;44:141–7. https://doi.org/10.1584/jpestics.D19-101 (PMID: 31530972).
Ohm RA, Feau N, Henrissat B, Schoch CL, Horwitz BA, Barry KW, et al. Diverse lifestyles and strategies of plant pathogenesis encoded in the genomes of eighteen Dothideomycetes fungi. PLoS Pathog. 2012;8:e1003037. https://doi.org/10.1371/journal.ppat.1003037 .
Rodriguez-Moreno L, Ebert MK, Bolton MD, Thomma BP. Tools of the crook—infection strategies of fungal plant pathogens. Plant J. 2018;93:664–74. https://doi.org/10.1111/tpj.13810 .
Horbach R, Navarro-Quesada AR, Knogge W, Deising HB. When and how to kill a plant cell: infection strategies of plant pathogenic fungi. J Plant Physiol. 2011;168:51–62.
Spraker JE, Wiemann P, Baccile JA, Venkatesh N, Schumacher J, Schroeder FC, et al. Conserved responses in a war of small molecules between a plant-pathogenic bacterium and fungi. mBio. 2018;9:E00820-18. https://doi.org/10.1128/mbio.00820-18 .
Venkatesh N, Keller NP. Mycotoxins in conversation with bacteria and fungi. Front Microbiol. 2019;10:403. https://doi.org/10.3389/fmicb.2019.00403 .
Mitchell NJ, Bowers E, Hurburgh C, Wu F. Potential economic loss to the US corn industry from aflatoxin contamination. Food Addit Contam Part A Chem Anal Control Expo Risk Assess. 2016;33:540–50. https://doi.org/10.1080/19440049.2016.1138545 .
Tannous J, Keller NP. Mycotoxins. In: Carroll KC, Pfaller MA, Landry ML, McAdam AJ, Patel R, Richter SS, et al., editors. Manual of clinical microbiology. 12th Edition. ASM Press; 2019. Chapter 129.
Moudgil V, Redhu D, Dhanda S, Singh J. A review of molecular mechanisms in the development of hepatocellular carcinoma by aflatoxin and hepatitis B and C viruses. J Environ Pathol Toxicol Oncol. 2013;32:165–75.
Lee RJ, Workman AD, Carey RM, Chen B, Rosen PL, Doghramji L, et al. Fungal aflatoxins reduce respiratory mucosal ciliary function. Sci Rep. 2016;6:33221. https://doi.org/10.1038/srep33221 .
Gratz SW. Do plant-bound masked mycotoxins contribute to toxicity? Toxins (Basel). 2017;9(p11):E85. https://doi.org/10.3390/toxins9030085 .
Van der Fels-Klerx HJ, Vermeulen LC, Gavai AK, Liu C. Climate change impacts on aflatoxin B1 in maize and aflatoxin M1 in milk: a case study of maize grown in Eastern Europe and imported to the Netherlands. PLoS ONE. 2019;14:e0218956. https://doi.org/10.1371/journal.pone.0218956 .
Assunção R, Martins C, Viegas S, Viegas C, Jakobsen LS, Pires S, et al. Climate change and the health impact of aflatoxins exposure in Portugal—an overview. Food Addit Contam Part A Chem Anal Control Expo Risk Assess. 2018;35:1610–21. https://doi.org/10.1080/19440049.2018.1447691 .
Moretti A, Pascale M, Logrieco AF. Mycotoxin risks under a climate change scenario in Europe. Trends Food Sci Technol. 2019;84:38–40. https://doi.org/10.1016/j.tifs.2018.03.008 .
Gruber-Dorninger C, Novak B, Nagl V, Berthiller F. Emerging mycotoxins: beyond traditionally determined food contaminants. J Agric Food Chem. 2017;65:7052–70. https://doi.org/10.1021/acs.jafc.6b03413 .
Smith MC, Madec S, Coton E, Hymery N. Natural co-occurrence of mycotoxins in foods and feeds and their in vitro combined toxicological effects. Toxins (Basel). 2016;8:94. https://doi.org/10.3390/toxins8040094 .
Meyer V. Genetic engineering of filamentous fungi—progress, obstacles and future trends. Biotechnol Adv. 2008;26:177–85. https://doi.org/10.1016/j.biotechadv.2007.12.001 .
Goffeau A, Barrell BG, Bussey H, Davis RW, Dujon B, Feldmann H, et al. Life with 6000 genes. Science. 1996;274(546):563–7.
Blattner FR, Plunkett G 3rd, Bloch CA, Perna NT, Burland V, Riley M, et al. The complete genome sequence of Escherichia coli K-12. Science. 1997;277:1453–62.
Saccharomyces Genome Database. https://www.yeastgenome.org/search?category=colleague&page=0 . Accessed 21 Feb 2020.
Gupta A, Xu W, Jaiswal P, Taylor C, Regala J. Domain informational vocabulary extraction experiences with publication pipeline integration and ontology curation. In: Proceedings of the 9th international conference on biological ontology (ICBO 2018), Corvallis, Oregon, USA. http://ceur-ws.org/Vol-2285/ICBO_2018_paper_43.pdf .
FungiDB. Fungal and oomycete genomics resources. https://fungidb.org/fungidb/ . Accessed 21 Feb 2020.
MycoCosm. The fungal genomics resource. https://mycocosm.jgi.doe.gov/mycocosm/home . Accessed 21 Feb 2020.
EnsemblFungi. https://fungi.ensembl.org/index.html . Accessed 21 Feb 2020.
ClinEpiDB. Clinical Epidemiology Resources. https://clinepidb.org . Accessed 21 Feb 2020.
Ruhamankaka E, Brunk BP, Dorsey G, Harb OS, Helb DA, Judkins J, et al. ClinEpiDB: an open-access clinical epidemiology database resource encouraging online exploration of complex studies. Gates Open Res. 2019;3:1661. https://doi.org/10.12688/gatesopenres.13087.1 .
HostDB. https://hostdb.org . Accessed 21 Feb 2020.
MicrobiomeDB. A microbiome resource. https://microbiomedb.org . Accessed 21 Feb 2020.
Basenko EY, Pulman JA, Shanmugasundram A, Harb OS, Crouch K, Starns D, et al. FungiDB: an integrated bioinformatic resource for fungi and oomycetes. J Fungi (Basel). 2018;4:E39.
Andersen MR. Elucidation of primary metabolic pathways in Aspergillus species: orphaned research in characterizing orphan genes. Brief Funct Genomics. 2014;13:451–5.
Cherry JM, Hong EL, Amundsen C, Balakrishnan R, Binkley G, Chan ET, et al. Saccharomyces genome database: the genomics resource of budding yeast. Nucleic Acids Res. 2012;40:700–5.
Schäpe P, Kwon MJ, Baumann B, Gutschmann B, Jung S, Lenz S, et al. Updating genome annotation for the microbial cell factory Aspergillus niger using gene co-expression networks. Nucleic Acids Res. 2019;47:559–69.
Steinbiss S, Silva-Franco F, Brunk B, Foth B, Hertz-Fowler C, Berriman M, et al. Companion : a web server for annotation and analysis of parasite genomes. Nucleic Acids Res. 2016;44:W29–34. https://doi.org/10.1093/nar/gkw292 .
Dunn NA, Unni DR, Diesh C, Munoz-Torres M, Harris NL, Yao E, et al. Apollo: democratizing genome annotation. PLoS Comput Biol. 2019;15:e1006790. https://doi.org/10.1371/journal.pcbi.1006790 .
VEuPathDB. https://veupathdb.org/ . Accessed 21 Feb 2020.
Vanegas KG, Jarczynska ZD, Strucko T, Mortensen UH. Cpf1 enables fast and efficient genome editing in Aspergilli. Fungal Biol Biotechnol. 2019;6:6. https://doi.org/10.1186/s40694-019-0069-6 .
Kwon MJ, Schütze T, Spohner S, Haefner S, Meyer V. Practical guidance for the implementation of the CRISPR genome editing tool in filamentous fungi. Fungal Biol Biotechnol. 2019;6:15. https://doi.org/10.1186/s40694-019-0079-4 .
Shapaval V, Brandenburg J, Blomqvist J, Tafintseva V, Passoth V, Sandgren M, et al. Biochemical profiling, prediction of total lipid content and fatty acid profile in oleaginous yeasts by FTIR spectroscopy. Biotechnol Biofuels. 2019;12:140. https://doi.org/10.1186/s13068-019-1481-0 .
Tolbert A, Ragauskas AJ. Advances in understanding the surface of lignocellulosic biomass via time-of-flight secondary ion mass spectrometry. Energy Sci Eng. 2017;5:5–20. https://doi.org/10.1002/ese3.144 .
Millet LJ, Aufrecht J, Labbé J, Uehling J, Vilgalys R, Estes ML, et al. Increasing access to microfluidics for studying fungi and other branched biological structures. Fungal Biol Biotechnol. 2019;6:1. https://doi.org/10.1186/s40694-019-0071-z .
Schmideder S, Barthel L, Friedrich T, Thalhammer M, Kovačević T, Niessen L, et al. An X-ray microtomography-based method for detailed analysis of the three-dimensional morphology of fungal pellets. Biotechnol Bioeng. 2019;116:1355–65. https://doi.org/10.1002/bit.26956 .
Schmideder S, Barthel L, Müller H, Meyer V, Briesen H. From three-dimensional morphology to effective diffusivity in filamentous fungal pellets. Biotechnol Bioeng. 2019;116:3360–71. https://doi.org/10.1002/bit.27166 .
Horta MAC, Thieme N, Gao Y, Burnum-Johnson KE, Nicora CD, Gritsenko MA, et al. Broad substrate-specific phosphorylation events are associated with the initial stage of plant cell wall recognition in Neurospora crassa . Front Microbiol. 2019;10:2317. https://doi.org/10.3389/fmicb.2019.02317 .
Weiss, B. The SEMI international standards program—history, successes and lessons learned to address compound semiconductor manufacturing challenges. In: 2006 International Conference on Compound Semiconductor Manufacturing Technology (CS MANTECH, 2006). p. 55–58. https://csmantech.org/OldSite/Digests/2006/2006%20Digests/4A.pdf . Accessed 5 Dec 2019.
Williams GW. The USDA Forest Service: the first century. 2005. https://www.fs.fed.us/sites/default/files/media/2015/06/The_USDA_Forest_Service_TheFirstCentury.pdf . Accessed 5 Dec 2019.
Prestemon JP, Wear DN, Foster MO. The global position of the U.S. forest products industry. US Dep Agric For Serv South Res Stn e-General Tech Rep. SRS-204, 2015:1–24.
Endy D. Foundations for engineering biology. Nature. 2005;438:449–53.
Mutalik VK, Guimaraes JC, Cambray G, Lam C, Christoffersen MJ, Mi QA, et al. Precise and reliable gene expression via standard transcription and translation initiation elements. Nat Methods. 2013;10:354–60.
Kahl L, Molloy J, Patron N, Matthewman C, Haseloff J, Grewal D, et al. Opening options for material transfer. Nat Biotechnol. 2018;36:923–7.
Streiff L. Enzyme toolkit made at Stanford helps make biotechnology globally accessible. Stanford News. 2019. https://news.stanford.edu/2019/11/22/enzyme-toolkit-makes-biotechnology-globally-accessible/ . Accessed 5 Dec 2019.
Better research through metrology. Nat Methods. 2018;15:395. https://doi.org/10.1038/s41592-018-0035-x .
Coxon CH, Longstaff C, Burns C. Applying the science of measurement to biology: why bother? PLoS Biol. 2019;17:e3000338.
Hernandez P. Joint initiative for metrology in biology. NIST 2016. https://www.nist.gov/jimb . Accessed 5 Dec 2019.
Perez R, Luccioni M, Gaut N, Stirling F, Kamakaka R, Adamala KP, et al. Enabling community-based metrology for wood-degrading fungi. bioRxiv. 2019. https://www.biorxiv.org/content/10.1101/815852v1 .
Hillson N, Caddick M, Cai Y, Carrasco JA, Chang ME, Curach NC, et al. Building a global alliance of biofoundries. Nat Commun. 2019;10:2040. https://doi.org/10.1038/s41467-019-10079-2 .
Kitney R, Adeogun M, Fujishima Y, Goñi-Moreno Á, Johnson R, Maxon M, et al. Enabling the advanced bioeconomy through public policy supporting biofoundries and engineering biology. Trends Biotechnol. 2019;37:917–20.
Gingko Bioworks. Gingko BioWorks—our foundries. 2019. https://www.ginkgobioworks.com/our-platform/ . Accessed 5 Dec 2019.
Ecovative Design LLC. Ecovative design—our foundry. 2019. https://ecovativedesign.com/ourfoundry . Accessed 5 Dec 2019.
Jessop-Fabre MM, Sonnenschein N. Improving reproducibility in synthetic biology. Front Bioeng Biotechnol. 2019;7:1–6.
Miles B, Lee PL. Achieving reproducibility and closed-loop automation in biological experimentation with an IoT-enabled lab of the future. SLAS Technol Transl Life Sci Innov. 2018;23:432–9.
Fredens J, Wang K, de la Torre D, Funke LFH, Robertson WE, Christova Y, et al. Total synthesis of Escherichia coli with a recoded genome. Nature. 2019;569:514–8. https://doi.org/10.1038/s41586-019-1192-5 .
Venet JE, Del Medico L, Wölfle A, Schächle P, Bucher Y, Appert D, et al. Chemical synthesis rewriting of a bacterial genome to achieve design flexibility and biological functionality. Proc Natl Acad Sci USA. 2019;116:8070–9.
Hutchison CA, Chuang R-Y, Noskov VN, Assad-Garcia N, Deerinck TJ, Ellisman MH, et al. Design and synthesis of a minimal bacterial genome. Science. 2016. https://doi.org/10.1126/science.aad6253 .
Richardson SM, Mitchell LA, Stracquadanio G, Yang K, Dymond JS, DiCarlo JE, et al. Design of a synthetic yeast genome. Science. 2017;355:1040–4.
Calles J, Justice I, Brinkley D, Garcia A, Endy D. Fail-safe genetic codes designed to intrinsically contain engineered organisms. Nucleic Acids Res. 2019;47:10439–51.
Download references
Acknowledgements
We thank the Industrial Platform of the EUROFUNG network for financially supporting the travel costs of the Think Tank meeting.
Author information
Authors and affiliations.
Chair of Applied and Molecular Microbiology, Institute of Biotechnology, Technische Universität Berlin, Gustav-Meyer-Allee 25, 13355, Berlin, Germany
Vera Meyer & Charlotte Steiniger
Institute of Integrative Biology, University of Liverpool, Biosciences Building, Crown Street, Liverpool, UK
Evelina Y. Basenko & Mark X. Caddick
TUM School of Life Sciences Weihenstephan, Technical University of Munich, Holzforschung München, Hans-Carl-von-Carlowitz-Platz 2, 85354, Freising, Germany
J. Philipp Benz
Department of Molecular Microbiology & Genetics, Institute of Microbiology & Genetics, Georg-August-Universität Göttingen, Grisebachstr. 8, 37077, Göttingen, Germany
Gerhard H. Braus
Syngenta, Jealott’s Hill International Research Centre, Bracknell, Berkshire, RG42 6EY, UK
Michael Csukai
Fungal Physiology, Westerdijk Fungal Biodiversity Institute & Fungal Molecular Physiology, Utrecht University Uppsalalaan 8, 3584 CT, Utrecht, Netherlands
Ronald P. de Vries
Department of Bioengineering, Stanford University, 443 Via Ortega, Stanford, CA, USA
Drew Endy & Rolando Perez
Department of Biotechnology and Biomedicine, Technical University of Denmark, 2800, Kongens Lyngby, Denmark
Jens C. Frisvad & Uffe H. Mortensen
Department Biology, Biotechnical Faculty, University of Ljubljana, Jamnikarjeva 101, 1000, Ljubljana, Slovenia
Nina Gunde-Cimerman
AB Enzymes GmbH, Feldbergstr. 78, 64293, Darmstadt, Germany
Thomas Haarmann
Department of Plant Pathology and Microbiology, Faculty of Agriculture, Food and Environment, The Hebrew University of Jerusalem, 76100, Rehovot, Israel
Yitzhak Hadar & Oded Yarden
Biotechnology Research, Production Strain Technology, Novozymes A/S, Krogshoejvej 36, 2880, Bagsvaerd, Denmark
Quorn Foods, Station Road, Stokesley, North Yorkshire, TS9 7AB, UK
Robert I. Johnson
Department of Medical Microbiology and Immunology, University of Wisconsin-Madison, Madison, 53706, USA
Nancy P. Keller
Department of Molecular Biology and Nanobiotechnology, National Institute of Chemistry, Hajdrihova 19, SI-1000, Ljubljana, Slovenia
Nada Kraševec
Institute of Biology Leiden, Molecular Microbiology and Biotechnology, Leiden University, Sylviusweg 72, 2333 BE, Leiden, The Netherlands
Arthur F. J. Ram
French National Institute for Agriculture, Food and the Environment, INRAE, UMR1163, Biodiversité et Biotechnologie Fongiques, Aix-Marseille Université, Marseille, France
Eric Record
MycoWorks, Inc, 669 Grand View Avenue, San Francisco, USA
Faculty of Science and Technology, Norwegian University of Life Sciences, Droebakveien, 31 1430, Aas, Norway
Volha Shapaval
Chr. Hansen A/S, Bøge Alle 10-12, 2970, Hørsholm, Denmark
Hans van den Brink
The University of Manchester, Manchester Institute of Biotechnology (MIB) & School of Natural Sciences, 131 Princess Street, Manchester, M1 7DN, UK
Jolanda van Munster
Department of Biology, Microbiology, Utrecht University, Padualaan 8, 3584 CH, Utrecht, The Netherlands
Han A. B. Wösten
You can also search for this author in PubMed Google Scholar
Contributions
VM hosted the EUROFUNG meeting and conceived the manuscript. All authors were involved in writing the final manuscript.
Corresponding author
Correspondence to Vera Meyer .
Ethics declarations
Ethics approval and consent to participate, consent for publication, competing interests.
The authors declare that they have no competing interests.
Additional information
Publisher's note.
Springer Nature remains neutral with regard to jurisdictional claims in published maps and institutional affiliations.
Supplementary information
Additional file 1: table s1..
Protein digestibility corrected amino acid (PDCAA) scores.
See Table 3 .
Rights and permissions
Open Access This article is licensed under a Creative Commons Attribution 4.0 International License, which permits use, sharing, adaptation, distribution and reproduction in any medium or format, as long as you give appropriate credit to the original author(s) and the source, provide a link to the Creative Commons licence, and indicate if changes were made. The images or other third party material in this article are included in the article's Creative Commons licence, unless indicated otherwise in a credit line to the material. If material is not included in the article's Creative Commons licence and your intended use is not permitted by statutory regulation or exceeds the permitted use, you will need to obtain permission directly from the copyright holder. To view a copy of this licence, visit http://creativecommons.org/licenses/by/4.0/ . The Creative Commons Public Domain Dedication waiver ( http://creativecommons.org/publicdomain/zero/1.0/ ) applies to the data made available in this article, unless otherwise stated in a credit line to the data.
Reprints and permissions
About this article
Cite this article.
Meyer, V., Basenko, E.Y., Benz, J.P. et al. Growing a circular economy with fungal biotechnology: a white paper. Fungal Biol Biotechnol 7 , 5 (2020). https://doi.org/10.1186/s40694-020-00095-z
Download citation
Received : 03 March 2020
Accepted : 23 March 2020
Published : 02 April 2020
DOI : https://doi.org/10.1186/s40694-020-00095-z
Share this article
Anyone you share the following link with will be able to read this content:
Sorry, a shareable link is not currently available for this article.
Provided by the Springer Nature SharedIt content-sharing initiative
Fungal Biology and Biotechnology
ISSN: 2054-3085
- General enquiries: [email protected]
Advertisement
The contribution of fungi to the global economy
- Open access
- Published: 12 July 2023
- Volume 121 , pages 95–137, ( 2023 )
Cite this article
You have full access to this open access article
- Allen Grace T. Niego 1 , 2 , 3 ,
- Christopher Lambert 4 , 5 ,
- Peter Mortimer 6 ,
- Naritsada Thongklang 1 , 2 ,
- Sylvie Rapior 7 , 8 ,
- Miriam Grosse 4 , 5 ,
- Hedda Schrey 4 , 5 ,
- Esteban Charria-Girón 4 , 5 ,
- Arttapon Walker 1 , 2 ,
- Kevin D. Hyde 1 , 2 , 9 , 10 &
- Marc Stadler ORCID: orcid.org/0000-0002-7284-8671 4 , 5
17k Accesses
17 Citations
120 Altmetric
Explore all metrics
Fungi provide ecological and environmental services to humans, as well as health and nutritional benefits, and are vital to numerous industries. Fermented food and beverage products from fungi are circulating in the market, generating billions of USD. However, the highest potential monetary value of fungi is their role in blue carbon trading because of their ability to sequester large amounts of carbon in the soil. There are no conclusive estimates available on the global monetary value of fungi, primarily because there are limited data for extrapolation. This study outlines the contribution of fungi to the global economy and provides a first attempt at quantifying the global monetary value of fungi. Our estimate of USD 54.57 trillion provides a starting point that can be analysed and improved, highlighting the significance of fungi and providing an appreciation of their value. This paper identifies the different economically valuable products and services provided by fungi. By giving a monetary value to all important fungal products, services, and industrial applications underscores their significance in biodiversity and conservation. Furthermore, if the value of fungi is well established, they will be considered in future policies for effective ecosystem management.
Similar content being viewed by others
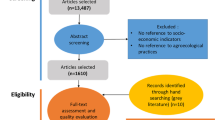
The socio-economic performance of agroecology. A review
Ioanna Mouratiadou, Alexander Wezel, … Paolo Bàrberi
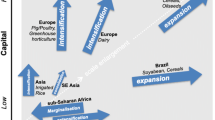
The future of farming: Who will produce our food?
Ken E. Giller, Thomas Delaune, … Martin K. van Ittersum
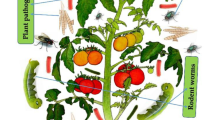
The use of microbial inoculants for biological control, plant growth promotion, and sustainable agriculture: A review
Ahmed S. M. Elnahal, Mohamed T. El-Saadony, … Khaled A. El-Tarabily
Avoid common mistakes on your manuscript.
Table of contents
Introduction.
Methods used to give monetary value to fungi
Data gathering
Market-based approach
Value Transfer Method (VTM)
Total Economic Value (TEV)
Real value of products and services from fungi
Value of carbon stocks being traded from forests
Industrial uses of fungi, medicines and pharmaceuticals.
Antibacterials
Penicillins
Cephalosporins
Fusidic acid
Pleuromutilin
Antimycotics
Echinocandins
Enfumafungin
Griseofulvin
Cardiovascular drugs
Immunosuppressive and immunomodulatory agents
Cyclosporin
Mycophenolic acid (mycophenolate mofetil)
Nematicides
Traditional Chinese medicines
Ophiocordyceps/Cordyceps
Food and beverages
Functional food and nutraceuticals
Agaricus bisporus
Hericium spp.
Lentinula edodes
Monascus purpureus
Ophiocordyceps sinensis
Fermented food
Baked goods
Alcoholic beverages and spirits
Non-alcoholic fermented beverages
Fungal food additives: organic acids
Citric acid
Fumaric acid
Gluconic acid
Itaconic acid
Lactic acid
Fungi based proteins
Food enhancers
Indonesian tempeh
Food colorants/pigments
Astaxanthin
Carotenoids
Monascus pigments
Commodities
Ergothioneine
Mycopesticides
Strobilurin fungicides
Mycorrhiza-based biofertilizers
Fungal enzymes
Galactosidase
Value of wild and cultivated mushrooms being traded for food
Emerging myco-based material industry, recreational fungal foraging, other potential biotechnological applications of fungi, bioremediation, monetary valuation of fungi, future perspectives/implication to policy makers, acknowledgements.
It is irrefutable that fungi play a pivotal role in any ecosystem. Detrital pathways are necessary for carbon and nutrient cycling (Liu et al. 2020a ). Fungi have very essential functions in addressing major global challenges. Wild mushrooms, especially if they can be cultivated, constitute a significant non-timber forest resource that provides various benefits, especially food and income for local communities in many parts of the world (Mortimer et al. 2012 ). They can be instrumental in improving resource efficiency as renewable substitutes for fossil resources. Moreover, they can be valuable sources of food, nutraceuticals, cosmeceuticals, amino acids, proteins, carbohydrates, vitamins, fats, and minerals (Ślusarczyk et al. 2021 ; Badalyan et al. 2022 ). They produce manifold bioactive compounds with medicinal, pharmaceutical, and industrial applications (Hyde et al. 2019 ; Niego et al. 2021 ; Galappaththi et al. 2022 ).
These numerous benefits of fungi are often overlooked, and the general population is often unaware of their value. Applied research has unfolded the role and potential of fungi in environmental services, such as bioremediation, mitigation of pollution, and as a resource for the development of drugs to combat various human diseases (Hyde et al. 2019 ). To fully utilize the potential of fungi, we need to understand their services rendered in ecosystems on a global scale. With the move towards a biobased circular economy (Meyer et al. 2020 ), we now consider it appropriate to make the world aware of the immense value of fungi for sustainable global development. Economic valuation is essential to create transparency with respect to the services rendered by fungi in ecosystems compared to other economic assets. This will provide clarity in political discussions, often dominated by monetary worth since ecosystem conversion appears to be more profitable than conservation. Economic criteria represent as well key elements during decision-making processes regarding conservation policies (Martin-Lopez et al. 2008 ; Takala et al. 2021 ).
The current review paper narrates the contribution of fungi in bio-economies. A greater understanding of the roles and value of fungi can be used to build global support for mycological appreciation. It will, in particular, raise policymakers' awareness of the importance of fungi, leading to future research and conservation efforts.
Methods used to give monetary value to fungal services
Data sources.
The available data were collected and extrapolated from published studies in scientific journals, databases, online market research, and reliable news and articles. Scientific journals were the major sources of reports for this paper. The published academic papers considered are from reliable journals, not listed in Beal’s list of potential predatory journals and publishers ( https://beallslist.net/ ). Key databases, which are listed below, provided reliable data for chemical/drug information (ChEBI 2022 ; Drugbank Online 2022a ; National Center for Biotechnology Information 2022a ; Special Chem 2022 ), industry reports and international trade reports (DEFRA 2006 ; OEC 2022a ; Tridge 2022a ), agricultural production (Food Data Central 2019 ; UNData 2022 ) and information on the populations (World Population Review 2022 ). Online market research reports are the main source for the market value of fungal products used in our study (Table 1 ). They provided reliable sources of data since they are based on literature analysis, annual reports, technical papers, news releases, white papers, conference papers, government publications, trade data and other literature for market studies. News on TV and social media, as well as articles in newspapers and magazines featuring economy news and market reviews can also provide the needed knowledge for fungal-marketed products, since many marketed values are not published in scientific journals (Table 1 ). However, we had experienced that some of the provider companies requested substantial fees when we asked them for details. This is why we were not able to add data on some of the included product lines.
Identification of economically important products and services of fungi
The first and most relevant method for this article is to identify and list the major individual products and services of fungi that contribute to the global economy. The marketable products and services are categorized into (i) wild and cultivated mushrooms traded for food, (ii) industrial uses of fungi (e.g. medicines and pharmaceuticals, food and beverages, and commodities), (iii) emerging biomaterial industries (e.g. myco-based materials, biofuels), (iv) marketable ecosystem services (carbon stock, fungal foraging). Only the top products, considered as those with a minimum global market value of at least a million dollars, were included. The valuation of the included products was taken from 2017 to 2022 or the latest available data point, respectively.
Literature reviews
Whenever possible, the monetary value of each product or service was identified based on reliable data sources, which were listed above. However, when there were not enough direct data available in the literature, monetary values were determined by using the value transfer method (Johnston and Wainger 2015 ).
Value transfer method
The value transfer or benefit transfer method refers to applying quantitative estimates of ecosystem service values from existing studies to another context. The values given in the literature are monetary estimates of benefits or costs. Data have been extrapolated from a study site and applied with adjustments to other policy sites. Value transfer is a continuum of approaches depending on the information available (Johnston and Wainger 2015 ). This method is applicable in giving monetary value to fungal traded services such as carbon stocking and recreational foraging.
Summation of values
Market values are categorized as definite value, product value and traded value, respectively. Definite value is the monetary value of a fungus itself (e.g., Agaricus bisporus and “ Cordyceps ”—i.e., Ophiocordyceps ; edible fungi: wild and cultivated fungi). Product value is the market value of the products derived from fungi or with the involvement of fungi in the production process (e.g., medicines and pharmaceuticals, fermented food and beverages, commodities and mycobased-material). Traded value is the market value from the services of fungi (e.g., carbon stock, recreational foraging). All these marketed values are combined to give an estimate of the monetary value of the fungal contribution to the global economy. All monetary values are given in US dollars (USD). For numerous industries that rely on fungi for the production processes, we have assumed the total industry value as the value of fungi, as it is impossible to disentangle the values of individual ingredients, and these products would not exist without the fungi involved.
Scope and limitation of the study
This study focusses on consumptive and marketed products and services rendered by fungi with direct effect on human well-being. Only those with a large impact on the global economy (minimum of USD 1 million) are included in the summation of values. Other ecosystem services and important roles in ecosystem functioning by fungi listed in Niego et al. ( 2023 ) with an indirect effect on humans will not be considered for valuation in this study, but future research is recommended to give them monetary value.
According to the Kyoto protocol, the Clean Development Mechanism (CDM) promotes afforestation and reforestation as carbon sequestration activities. It was expected to be the most effective and easily measurable method for impounding carbon as biomass above and below the ground. During the negotiation of the Kyoto Protocol under the United Nations Framework Convention on Climate Change, 38 countries were required to reduce greenhouse gas (GHG) emissions by at least 5.2% below the 1990 levels. This goal was achieved by reducing greenhouse gas emissions or increasing greenhouse gas sinks (Tulpule et al. 1998 ; Grubb et al. 1999 ). A carbon price is an instrument used to capture the cost of greenhouse gas emissions, such as the damage caused to the environment. Governments and business sectors increasingly agree that carbon pricing plays a vital role in the transition to a decarbonized economy. In addition to charging emitters for carbon dioxide (CO 2 ) emissions, carbon pricing also provides incentives to individuals who emit less (Barron et al. 2019). Global carbon trading was valued at €760 billion (USD 817 billion) in 2021 with EU emission allowances above €80/t (USD 86/t) (Refinitiv 2022 ).
Carbon stock in forests can be found as 31% biomass and 69% in the soil (IPCC 2005 ). In the study by Clemmensen et al. ( 2013 ), it was stated that 50–70% of sequestrated carbon in the soil was derived from roots and root-associated microorganisms such as mycorrhizal fungi. Approximately 85% of the terrestrial plants form mycorrhizal associations with fungi. The ectomycorrhizal and ericoid mycorrhizal plants associates are the majority of trees (92%) and shrubs (85%), while arbuscular mycorrhizal plant associates are mostly herbaceous (50%) (Soudzilovskaia et al. 2020 ).
Different types of mycorrhizal association present in forest ecosystems can affect the global distribution of carbon stock. Soudzilovskaia et al. ( 2019 ) estimated the storage of carbon in arbuscular (AM), ectomycorrhizal (EcM) and ericoid (ER) mycorrhizal vegetation in above-ground biomass as 241 ± 15, 100 ± 17, and 7 ± 1.8 billion metric tons, respectively. We assumed that the EcM and ER vegetations are in the forest, since these groups of fungi are mostly associated with trees. Based on the recent study of Edy et al. ( 2022 ) AM fungi are also present in 75% of tropical forests. The monetary values of carbon sequestered by different mycorrhizal vegetation were estimated by multiplying the values by the carbon emission allowance of USD 86/t of carbon (Refinitiv 2022 ) (Table 2 ). The total monetary value of the carbon stock from above-ground biomass by mycorrhizal vegetation worldwide is USD 24,768 billion, equivalent to 31% of the carbon stock in the forest (IPCC 2005 ). From this value, the total value of carbon stock in the forest is estimated to be USD 79,897 billion, of which USD 55,129 billion is contributed by soil carbon of 69% (IPCC 2005 ). Since 50–70% of the sequestered carbon in the soil is associated with root-associated microorganisms, we can roughly assume that the value of the carbon stock in the soil related to mycorrhizae is at least USD 27,565 billion. Thus, the total market value of the carbon stock associated with mycorrhizae is USD 52,333 billion .
Fungi and the welfare obtained from them have been instrumental in the development of essential industrial processes worldwide. Among others, the secondary metabolites produced by these organisms represent a valuable resource in the development of medicines and pharmaceuticals. Fungal-based processes and fungal enzymes also play a key role in the production of food and beverages, as well as different commodities. The market value of these products steadily increases as the years go by. This chapter will discuss the different products and their market values, based on the available data.
Fungi have been explored for their medicinal value over thousands of years (Zhang et al. 2015 ). Aside from nutritional benefits, their ability to produce secondary metabolites makes them an important source for innovative chemistry, which led to the development of several important pharmaceuticals that have been beneficial for the advance of human civilization over time. While natural products and secondary metabolites in general have resulted in many drugs for the treatment of various diseases (Newman and Cragg 2020 ), fungal metabolites have been only developed for part of these indications, as amply summarized by Bills and Gloer ( 2016 ). For instance, contrary to what is stated in many reviews that have been published on the subject, there are no ethical anticancer drugs from fungi on the market. Taxol, for instance, is exclusively produced using plants as sources and the recent classification of the fungal “endophyte” that was reported to be able to produce the metabolite as a member of the Basidiomycota (Cheng et al. 2022 ) raises serious concerns as to whether fungi can produce this metabolite at all. In any case, fungal metabolites have given rise to the development of several drugs that gained a rather high commercial value in other indications, above all as anti-infectives, but even cardiovascular and immunomodulatory agents. However, even the discovery of some blockbuster drugs like cyclosporin has been attributed to their ability to exert antibiosis, and their true potential was discovered later when they were tested in more sophisticated bioassays. Growth inhibitory or toxic properties of fungal secondary metabolites have regularly been linked with ensuring their survival in competitive natural environments (Keller 2019 ). Of the fungal natural product—derived drugs found on the market, the majority were originally isolated from species of the Ascomycota, while a few are derived from Basidiomycota (Bills and Gloer 2016 ; Sandargo et al. 2019 ). Compared to the vast number of described compounds, only a handful have been followed up upon to be further developed into marketable drugs (Mapook et al. 2022 ) (Table 3 ). Nevertheless, the secondary metabolite-inspired drugs that are on the market have been proven indispensable for modern medicine and managing diseases in general, displaying important sources of economic revenue.
Penicillins are the first β-lactam antibiotics explored for their medicinal value and are still one of the most widely used antibiotic agents to fight bacterial infectious diseases. They were first described from Penicillium rubens (Houbraken et al. 2011 ) but also reported from other species such as P. chrysogenum and P. griseofulvum (Laich et al. 2002 ). Penicillins are still in use to treat a wide range of bacterial infections (Lobanovska and Pilla 2017 ). Aside from the formulated naturally occurring derivatives penicillin G and V, other semi-synthetic derivatives among the vast diversity of analogues are Amoxicillin, Ampicillin, Piperacillin, Dicloxacillin, Nafcillin and Oxacillin (Wright 1999 ). The global Penicillin market size is estimated to be worth USD 1.96 billion in 2022 and is forecasted to increase to USD 2.4 billion by 2028 with a compound annual growth rate of 3.8% (Market Watch 2022a ).
Cephalosporins are another class of β-lactam antibiotics originally isolated from strains of the genus Acremonium. Following its first report by Newton and Abraham ( 1955 ), a semi-synthetic derivative of cephalosporin C named cephalothin reached the market in 1964 (Asbel and Levison 2000 ). Since then, in total five generations of cephalosporin-derived antibiotics were developed, further improving upon its selectivity against drug resistant bacteria (Lin and Kück 2022 ). Among the indications are skin infections, with and without multi drug resistant bacteria and meningitis, to name a few (Lin and Kück 2022 ). The global cephalosporin market size was valued at USD 18.7 billion in 2022 , and it is expected to reach USD 22.3 billion by 2028, exhibiting a CAGR of 2.83% during 2023–2028 (Imarc 2022a ).
First generation cephalosporins are commonly used to manage skin and soft tissue infections caused by susceptible strains of Staphylococcus aureus and group A Streptococcus . Those include cefazolin, a parenteral formulation, and cephalexin, an oral equivalent, among others (Lin and Kück 2022 ).
Second generation cephalosporins have a better performance against Gram-negative bacterial infections than the first-generation derivatives and are used to treat urinary and tract infections, and skin and soft tissue infections. A variety of orally administered second-generation agents (cefaclor, cefprozil, loracarbef, cefpodoxime) are commonly used in the outpatient management of sinopulmonary infections and otitis media (Lin and Kück 2022 ).
Third generation cephalosporins are typically used for serious pediatric infections, including meningitis and sepsis (Lin and Kück 2022 ).
Fourth generation cephalosporins include, e.g. cefepime, which is active against Pseudomonas aeruginosa and retains good activity against methicillin-susceptible staphylococcal infections (Lin and Kück 2022 ).
An example for Fifth generation cephalosporins is ceftaroline, the active metabolite of the prodrug ceftaroline fosamil. This is a broad-spectrum cephalosporin with bactericidal activity against resistant Gram-positive bacteria, including Methicillin-resistant Staphylococcus aureus (MRSA), and common Gram-negative pathogens (Lin and Kück 2022 ). The development of cephalosporins is a prime example of how a basic structure derived from natural sources can be gradually improved via semisynthesis and medicinal chemistry approaches, finally leading to broad spectrum antibiotics. However, this development has taken several decades of hard work.
In this context, it is also worthwhile to mention the carbapenems , which are also β-lactam antibiotics but were discovered from Streptomyces species, which are Gram-positive Actinobacteria. Olivanic acids were the first isolated carbapenems from S. clavuligerus in a screening campaign for β-lactamase inhibitors (Brown et al. 1976 ), but the compound family only went into clinical relevance with the isolation of thienamycin from S. cattleya , which exhibited superior physicochemical properties (Kahan et al. 1979 ; Papp-Wallace et al. 2011 ). Carbapenems combine two activities as being able to bind to the same target as other β-lactams, in addition to their inherent property to act as a β-lactamase inhibitor. The first carbapenem to enter the market was the semi-synthetic analogue imipenem (Miyadera et al. 1983 ) in 1985, which was followed by other synthetic analogues like meropenem (Sunagawa et al. 1990 ), biapenem (Ubukata et al. 1990 ), ertapenem (Sundelof et al. 1997 ), doripenem (Tsuji et al. 1998 ), and panipenem (Neu et al. 1986 ). These compounds are used to treat a broad band of bacterial infections, including complicated nosocomial bacteriosis, or infections caused by multi-drug resistant staphylococci and Pseudomonas (Papp-Wallace et al. 2011 ). Due to their inability to pass the gastrointestinal tract, they are commonly administered intravenously. However, the new drug tebipenem is currently in clinical trials as the first orally available carbapenem (Jain et al. 2018 ). The global carbapenem market was valued at USD 3.9 billion in 2021 and is expected to exhibit a growth rate (CAGR) of 4.5% during 2022–2030 (Polaris Market Research 2022 ).
Fusidic acid is a bacteriostatic triterpene derived from the fungus Ramularia coccinea (Godtfredsen et al. 1962 ; as Fusidium coccineum ) and used as a topical medication for the treatment of skin infections with Gram-positive bacteria (Fernandes 2016 ). It acts as a bacterial protein synthesis inhibitor by binding to the elongation factor G (EF-G) bound to ribosomes. This effectively prevents the translocation of the newly assembled polypeptide chain during protein synthesis. Recycling of ribosomal subunits is thus inhibited after completion of transcription (Gao et al. 2009 ) The global fusidic acid market value was estimated at USD 171.89 million in 2021 with projection to reach up to USD 271.84 million by 2028, exhibiting a CAGR of 6.77% during the forecast period (Market Watch 2022b ).
Pleuromutilin and its derivatives are antibacterials produced by Basidiomycota that inhibit protein synthesis in bacteria by inhibiting peptide bond formation through binding to the 50S subunit of ribosomes (Paukner and Riedl 2017 ). For details we refer to the recent review by (Mapook et al. 2022 ). The pleuromutilin derived compounds tiamulin and valnemulin covered 2.5% of total sales of antimicrobials used for food producing animals (European Medicines Agency 2013). Lefamulin, a new pleuromutilin antibiotic with the brand name XENLETA™, which is used for the treatment of community-acquired pneumonia (CAP), is expected to peak sales of USD 267 million by the end of 2025 (Joseph 2019 ). As this drug is fairly new to the Pharma market and has a molecular target that is not addressed by the conventional antibiotics, a substantial increase in the sales is to be expected in the coming years (Fig. 1 ).
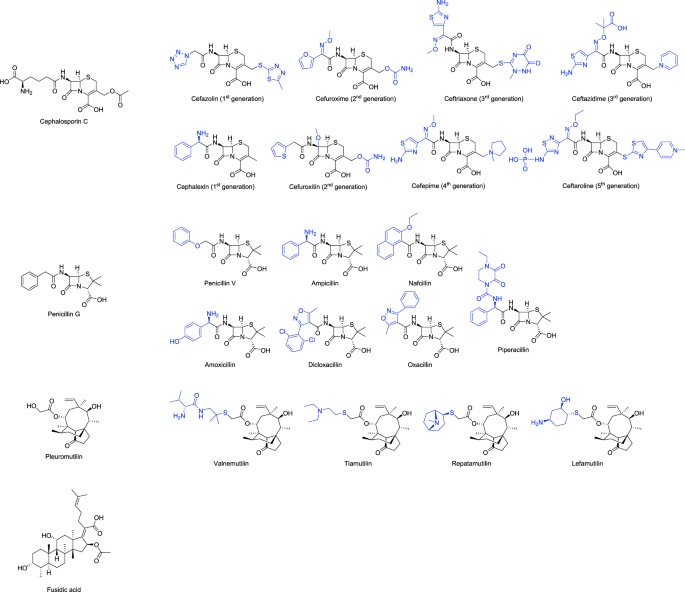
Chemical structures of antibacterial drugs
Echinocandins are a group of cyclic non-ribosomal lipopeptides reported from Ascomycota of the classes Leotiomycetes and Eurotiomycetes (Hüttel 2021 ). Currently, there are three semi-synthetically modified drugs, derived from echinocandin B (producer: Aspergillus delacroxii ; Benz et al. 1974 ), pneumocandin A 0 ( Glarea lozoyensis ; Schwartz et al. 1989 ) and FR901379 ( Coleophoma empetri ; Iwamoto et al. 1994 ) on the market. All three drugs, anidulafungin , caspofungin and micafungin are used to treat and prevent invasive fungal infections including candidemia, abscesses, esophageal candidiasis, and certain other invasive Candida infections (Hüttel 2021 ). Furthermore, these pharmaceutics are used as prophylaxis for Candida infections during stem cell transplantation. Antifungal effect relies on the inhibition of the synthesis of β-(1,3)- d -glucan, an integral component of the fungal cell wall (Sawistowska-Schröder et al. 1984 ). The global market size for echinocandins was valued at USD 515.20 million in 2021 and is expected to reach USD 796.69 million by 2029, with a CAGR of 5.6% (Data Bridge Market Research 2022 ).
Enfumafungin is a triterpene glycoside and hemiacetal derived from the endophytic fungus Hormonema sp. (Peláez et al. 2000 ). It is a potent antimycotic which, similarly to the echinocandins, inhibits the biosynthesis of β-(1,3)- d -glucan (Jallow and Govender 2021 ). Its semi synthetic derivative Ibrexafungerp was the first-in-class triterpenoid antifungal drug with broad spectrum antifungal activity (Ghannoum et al. 2020 ) that has been approved for the treatment of vulvovaginal candidiasis (VVC) and only recently entered the market (Espinel-Ingroff and Dannaoui 2021 ). It is sold under the brand name Brexafemme® approved by the FDA in June 2021 with net revenues of USD 0.7, 1.3, and 1.16 million in the first three quarters of 2022 respectively (GlobeNewswire 2022 ; Scynexis 2022 ).
Griseofulvin is a secondary metabolite produced by Penicillium spp. and was first discovered from P. griseofulvum (Oxford et al. 1939 ). It has been developed as an orally available, fungistatic drug used to treat superficial fungal skin infections such as tinea capitis and pedis (Gupta et al. 2017 ). The onychomycosis treatment market value exceeded USD 4.2 billion in 2020 and expected to reach USD 6.2 billion in 2027 (Global Market Insights 2022a ). Although there is no market value reported in the literature for griseofulvin, it is worth mentioning this important product because it is one of the most valuable treatments for onychomycosis on the market (Fig. 2 ).
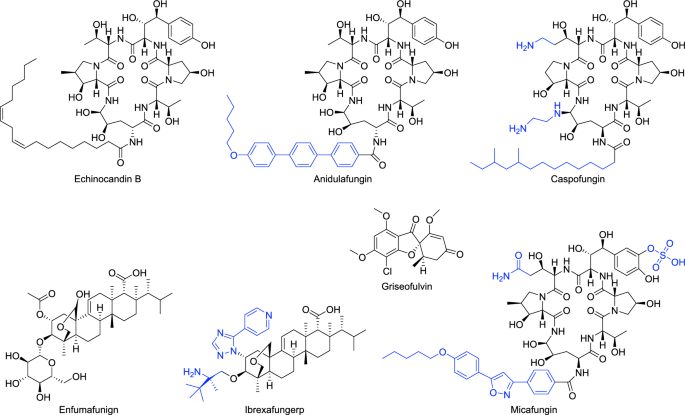
Chemical structures of antimycotic drugs
Statins are prescribed for their cholesterol-lowering capacity to prevent mortality linked towards cardiovascular diseases, such as coronary artery disease and stroke (Endo 2008 ). Among the marketed statins are pravastatin , the semi-synthetic product of compactin (or mevastatin) isolated from Penicillium citrinum (Endo et al. 1976 ), lovastatin and its semi-synthetic derivative simvastatin (Pedersen and Tobert 2004 ). In addition, some of the synthetic statins inspired by nature introduced to the market are fluvastatin, atorvastatin, rosuvastatin and pitavastatin (Endo 2010 ). Lovastatin was concurrently isolated from Aspergillus terreus and Monascus ruber (reported as Monacolin K, Endo 1979 ) and was one of the first statins to be extensively used for its cholesterol-lowering ability (Steinberg 2006 ) and even discussed as auxiliary drugs in treatment regiments against cancer (Jiang et al. 2021 ). The global statins market size was valued at USD 14.3 billion in 2021 , and was expected to reach USD 17.5 billion by 2027, with a CAGR of 3.4% during 2022–2027 (Imarc 2022b ) (Fig. 3 ).
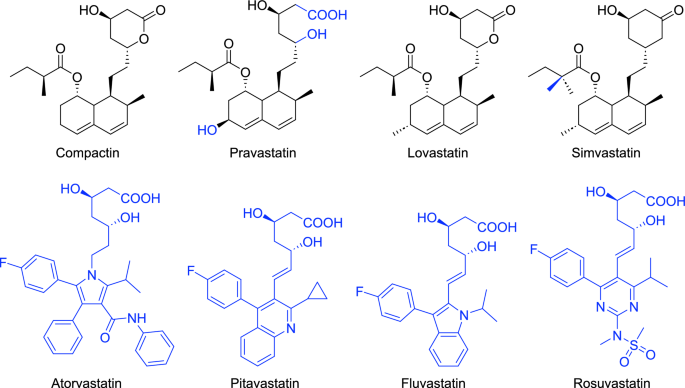
Chemical structures of cardiovascular drugs
Cyclosporin A represents the first calcineurin inhibitor, which was reported from the hypocrealean soil fungus Tolypocladium inflatum (originally identified as “ Trichoderma polysporum ” by Rüegger et al. 1976 ) and entered the market in 1983 (Survase et al. 2011 ). This substance was shown to exhibit a better bioactivity profile in terms of its immunosuppressive activities as compared to other synthetic drugs on the market, which turned out to be a game changer in the field of transplantation medicine (Demain and Sanchez 2009 ; Survase et al. 2011 ). The market sales of cyclosporin A totaled to USD 1.5 billion in 2009. Recently, cyclosporine was valued at USD 1.99 billion in 2021 , and it is projected to reach the value of USD 6.08 billion in 2030 (Verified Market Research 2022a ).
Fingolimod is an immunomodulating drug developed from myriocin. It was first reported from Melanocarpus albomyces (previous name: Myriococcum albomyces ; cf. Kluepfel et al. 1972 ). The semi-synthetic derivative was shown to modulate the sphingosine 1-phosphate receptor, which is exploited to treat patients with a relapsing–remitting form of multiple sclerosis (MS, Volpi et al. 2019 ). It is sold since 2010 (Thomas et al. 2017 ) under the brand name Gilenya (Drugbank Online 2022b ). Gilenya has a market share of USD 79,411 per 1000 Medicare beneficiaries in 2014 (San-Juan-Rodriguez et al. 2019 ) with annual sales worth USD 3.34 billion in 2018 (Miller 2019 ).
Mycophenolic acid is derived from Penicillium brevicompactum and originally reported by Gosio ( 1893 ) as the first fungal antibiotic. However, it was only much later that systemic studies and semi-synthetic derivatization improved its toxic side effects to enable its usage as an immunosuppressant for organ transplantations as of starting from 1995 (Lipsky 1996 ). The compound itself, however, is toxic and commonly prescribed as its formulated prodrug, mycophenolate mofetil. Upon release of its active component (mycophenolic acid), it inhibits the inosine monophosphate dehydrogenase, which is required for the de novo synthesis of guanosine (Lenihan and Tan 2020 ). The global market value of mycophenolate mofetil was USD 1581.34 million in 2022 (Market Watch 2023a ). Mycophenolate sodium was later introduced to the market as an enteric-coated slow-release formulation of mycophenolic acid to decrease the gastrointestinal side effects of mycophenolate mofetil (Lenihan and Tan 2020 ). This drug has been in discussion for its application in other medical conditions associated with an apparently hyperactive immune systems as well, such as psoriasis (Bentley 2000 ).
Mizoribine is an immuno-suppressive sold in Japan, Korea, and China and commonly used for treatment of renal diseases and prevention of renal transplants rejection (Kawasaki 2009 ). The compound was first isolated from Penicillium brefeldianum (Mizuno et al. 1974 ) and came on the market in 1984, however, limitations concerning its efficacy allegedly limits its use worldwide (Kawasaki 2009 ). Mizoribine is estimated to be worth several million USD in 2021, however, the exact value was not available to us (Fig. 4 ).
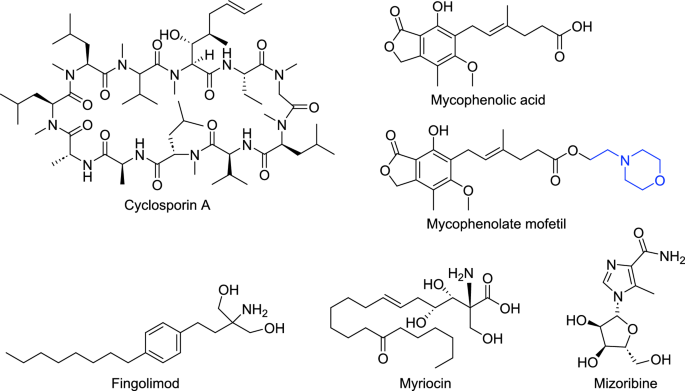
Chemical structures of immunosuppressive and immunomodulatory agents
Emodepside has been the first drug on the market that is derived from a secondary metabolite produced by a fungal endophyte (Sasaki et al. 1992 ; Scherkenbeck et al. 2002 ; Helaly et al. 2018 ). Molecular Data in patents already pointed towards this fungus, which was isolated from a tea plant in Japan, being a member of the genus Rosellinia (Harder et al. 2011 ). However, it was only shown recently that ascospore-derived isolates of the latter xylariaceous genus can indeed be linked with the production of the nematicidal PF1022 derivatives (Wittstein et al. 2020 ). The semi-synthetic derivative is marketed under Emodepside and used as an anti-helminthic drug in veterinary medicine (Willson et al. 2003 ). Currently, attempts are ongoing to develop the compound as a remedy for human pathogenic nematode infections. In this sense, emodepside appears as a promising candidate with a novel mode of action against human river blindness (onchocerciasis), a disease caused by the filarial worm Onchocerca volvulus , and which treatment relies on the natural product ivermectin (Krücken et al. 2021 ). Recently, it has been validated that emodepside exhibits good in vitro efficacy against microfilarial, third larval, fourth larval, and adult stages of various filarial genera and species (Hübner et al. 2021 ). There are no data available on the current market value of emodepside. The compound is only administered with another synthetic anthelmintic agent, praziquantel, in veterinary medicine (Fig. 5 ).
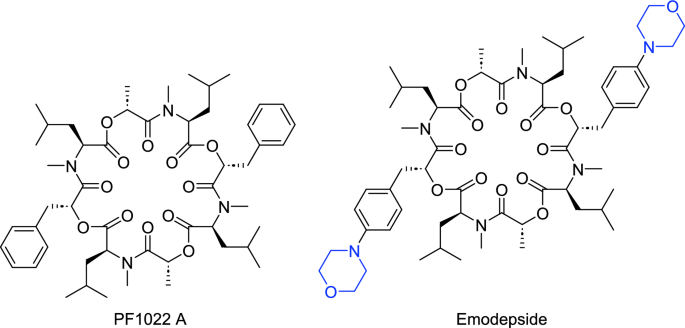
Chemical structures of nematicidal agents
Traditional Chinese medicines (TCM)
Traditional Chinese medicine (TCM) has existed for almost 5000 years and was historically used by the Chinese to combat diseases. Until now, interest and acceptance of TCM continue to grow worldwide (Lin et al. 2018 ). Fungi have always been part of TCM, and the most explored species belong to the genera Ophiocordyceps, Cordyceps, and Ganoderma (Paterson 2006 , 2008 ).
Ophiocordyceps/Cordyceps preparations are commonly used in traditional Chinese medicines in China, Japan, Korea, and other eastern Asian countries (Zhu et al. 1998 ). They were used in China for at least 2000 year (Zhu et al. 1998 ). Ophiocordyceps sinensis , Cordyceps militaris, Cordyceps guangdongensis, and Isaria cicadae are the most explored and commercialized “ Cordyceps ” fungi in China (Zhu et al. 1998 ; Zhang et al. 2014 ). There is a huge demand in Chinese markets for O. sinensis, with an annual yield of 100–150 tons (of which approximately 100 tons are produced in China). The market price is USD 8 to USD 25 each or USD 25,000 to 60,000 per kilogram (Zha et al. 2018 ). The national annual value of natural Chinese Cordyceps is estimated to be over 10 billion RMB (USD 1.5 billion) in 2004 (Dong et al. 2015 ).
Ganoderma -based products are marketed in many Asian, European and North American countries, although South Asian countries such as China, Japan, Korea, Malaysia and Singapore are the main producers of the food products (Chang and Miles 2004b ). The annual global market turnover of Ganoderma -based products was approximately USD 2.16 billion in 2002 (Lai et al. 2004 ). In 2019, the global market value of Ganoderma- based products was USD 3.1 billion , and projected to reach USD 5.1 billion in 2027 (Allied Market Research 2022a ).
Fungi have long been explored due to their medicinal value (De Silva et al. 2013 ; Hyde et al. 2019 ; Badalyan and Rapior 2021 ) and many fungal products are produced as nutraceuticals, including cosmeceuticals (Bandara et al. 2015 ; Badalyan et al. 2022 ). Nutraceuticals are a group of products from edible sources, proven to be safe for consumption and can be considered a supplement to effective pharmacological treatment (Niego et al. 2021 ). Fungal products are being consumed as functional foods, dietary supplements, and sources of bioactive compounds with a range of medicinal applications (Hyde et al. 2019 ; Badalyan et al. 2021 ). Some of the most explored genera are Agaricus, Hericium, Lentinula, and Ophiocordyceps (Valverde et al. 2015 ; Hyde et al. 2019 ; Niego et al. 2021 ). The reported bioactivities include antibacterial, antidiabetic, antifungal, antihypercholesterolemial, antioxidant, antiparasitic, antitumor, antiviral, cardiovascular, hepatoprotective, immunomodulatory and radical scavenging effects (Hyde et al. 2019 ; Zeb and Lee 2021 ; Niego et al. 2021 ). The bioactive principles causative for those effects are polysaccharides, proteins (El Enshasy and Hatti-Kaul 2013 ), glycoproteins (Cohen et al. 2014 ), phenolic compounds (Gupta et al. 2018 ; Hamwenye et al. 2022 ), ergosterol (Diallo et al. 2020 ) and other terpenoids, or unsaturated fatty acids (Galappaththi et al. 2022 ). The health-promoting properties of many fungi are the driving force for increasing their market value and incorporation into the nutraceutical industry (Niego et al. 2021 ). The following are economically important fungi explored in the nutraceutical and functional food industries (Table 4 ).
The bioactivities of Agaricus bisporus have been quite established. It is one of the most explored mushrooms for nutraceuticals (Gopalakrishnan et al. 2005 ). Agaricus bisporus was valued globally at USD 16.69 billion in 2022 (Market Data Forecast 2022a ).
Hericium species (and in particular H. erinaceus ) are highly valued for their medicinal properties. China is the main exporter of Hericium with a market value of USD 270 million, followed by Italy (USD 90.97 million) and Poland (USD 65.83 million) in 2021 (Tridge 2022a ). The global export value of Hericium was USD 963.4 million and the import value was USD 978.83 million in 2021 (Tridge 2022b ).
The nutraceutical properties of Lentinula edodes have been well documented (Liu et al. 2020b ; Wang et al. 2020 ; Raghoonundon et al. 2021 ). The global market value of Lentinula (Shiitake) is USD 4.31 billion in 2022 and is expected to reach USD 4.7 billion in 2030 at a CAGR of 8.3% (The Brainy Insights 2022 ).
Red yeast rice was created by fermenting Monascus purpureus on white rice. In traditional Chinese medicine, the powdered yeast-rice mixture has been utilized as a staple food in Asia (Nguyen et al. 2017 ). For heart disease and high cholesterol, people consume red yeast rice orally as a supplement. (Dufossé 2019 ). The cholesterol-lowering properties and other beneficial effects that have been proven for the product is, however, not due to the pigments (Li et al. 1998 ). The substance monacolin K (see above under “statins”) is one of them (Nguyen et al. 2017 ). The red yeast rice had a market value of USD 380.6 million in 2022 (Persistence Market Research 2022 ).
Ophiocordyceps sinensis is one of the most well-known and most expensive fungal species worldwide, with a market price that reached up to USD 60,000/kg in 2015 (Lei et al. 2015 ). Various nutraceutical products have been derived from Ophiocordyceps sinensis. In China, more than 1000 nutraceutical products are prepared from this species (Rakhee et al. 2021 ). The global market value of O. sinensis was around USD 5–11 billion in 2018 (He 2018 ).
The market value of these economically important mushrooms, however, was mainly due to the fact that they are also food sources and not solely functional food or nutraceuticals, particularly A. bisporus and L. edodes which are also some of the most cultivated mushrooms for food, thus will not be included in the summation for functional food to avoid double inclusion. By definition, functional food refers to food or food ingredients providing health benefits beyond basic nutrition (Colorado State University Extension 2023). The global functional mushroom market was then valued at USD 12.34 billion.
Fungi are beneficial to humans both industrially and commercially, especially in food and beverage production (Koul and Farooq 2020 ). Fermented foods and alcoholic beverages have long been an essential part of the human diet around the world. These foods are often well preserved with high nutrient content such as proteins, vitamins, minerals, and other nutrients (Tamang et al. 2020 ). The availability of nutrients in fermented foods and beverages is mainly due to the fermentation process performed by living microorganisms such as bacteria and fungi (Rezac et al. 2018 ). These microorganisms may also improve gastrointestinal health and provide other health benefits as probiotics (Rezac et al. 2018 ) that contribute to the popularity of fermented products (Fortune Business Insight 2022a ). Saccharomyces cerevisiae , Candida spp., Debaryomyces spp. and Wickerhamomyces anomalus are the most common yeasts associated with traditional fermentations of fermented foods and beverages (Bhalla and Savitri 2017 ). Saccharomyces cerevisiae is the yeast species most frequently involved in alcoholic fermentation and is also used in most other fermented products (Walker and Stewart 2016 ). Fermentation of foods and beverages changes the flavor and taste of the product, as well as its nutritional value and digestibility (Fortune Business Insight 2022a ). Table 5 lists some economically important fermented foods and beverages along with their global market value.
Baked goods are an essential part of human food. Saccharomyces cerevisiae is a very important fungus in the food production industry and is known as Baker's Yeast. It converts sugars and starches into alcohol and carbon dioxide during the fermentation process. Saccharomyces cerevisiae has been bred into various strains which produce significantly more CO 2 , and thus can achieve faster fermentation than wild type strains (Lahue et al. 2020 ). The global value of the market for bakery products reached USD 497.50 billion in 2022 (Imarc 2023 ). The top exporters are Germany (USD 4.3 billion), Canada (USD 3.14 billion), Italy (USD 2.73 billion), France (USD 2.28 billion) and the USA (USD 2.04 billion) (OEC 2022b ).
Cheese is a widely consumed dairy product with numerous varieties such as cheddar, feta, gouda, parmesan and camembert, produced in different parts of the world. Filamentous fungi and yeasts are used in the production of different kinds of cheese (Metin 2018 ; Chourasia et al. 2021 ). Filamentous fungi that thrive in cheese include Aspergillus, Cladosporium, Geotrichum, Mucor, Penicillium , and Trichoderma (Hymery et al. 2014 ) . There are two categories of mold-ripened cheeses: internally ripened and surface-ripened. The most well-known examples of internally ripened cheeses are the blue cheeses caused by Penicillium roqueforti creating blue veins, as the name suggests. The ripening of the famous Camembert-type soft cheeses relies on Penicillium camemberti (Lessard et al. 2014 ; Ropars et al. 2020 ) . Another example is Norwegian Gamalost with its ripening caused by Mucor with a yellow–brown color. Surface-ripened cheeses can be hard, semi-hard, and soft. The most common examples of hard surface-ripened cheeses are French Cantal, Salers, and Rodez, which involve species of Scopulariopsis or Sporendonema casei . The Saint Nectaire semi-hard cheese uses Mucor spp. and Bisifusarium domesticum as ripening molds (Zhang and Zhao 2010 ; Zhang et al. 2012 ; Metin 2018 ).
The global value of the cheese market was USD 231.65 billion in 2022 (The Business Research Company 2023 ). The top exporters were Germany (USD 4.79 billion), Netherlands (USD 4.11 billion), Italy (USD 3.57 billion), France (USD 3.49 billion), and Denmark (USD 1.71 billion) (OEC 2022c ). Blue cheese production had a total net trade of USD 624 million in 2020 wherein the top exporters are Italy (USD 185 million), Denmark (USD 122 million), France (USD 121 million, Germany (USD 94.8 million), and the United Kingdom (USD 17.1 million) (OEC 2022a ).
Alcoholic beverage and spirits
Yeasts, especially Saccharomyces cerevisiae, play a vital role in the production of fermented beverages. They are involved in the production of all alcoholic beverages and the selection of suitable yeast strains is essential not only to maximize alcohol yield, but also to maintain beverage sensory quality (Walker and Stewart 2016 ). Saccharomyces cerevisiae is the most common yeast used for many years in the production of beverages associated with the fermentation process (de Almeida Silva Vilela et al. 2020 ). Fermented beverages are made by yeast by converting sugar together with other essential nutrients such as amino acids, minerals, and vitamins into alcohol and carbon dioxide (primary fermentation metabolites) and other chief secondary metabolites (Walker and Stewart 2016 ; Vilela 2019 ). Secondary metabolites produced by yeast act as important flavor congeners of beverages that influence the final taste and aroma of alcoholic beverages such as beer and wine, as well as other distilled beverages such as brandy, rum, and whiskey (Walker and Stewart 2016 ). Yeasts are also involved in the production of white spirits such as gin and vodka (Pauley and Maskell 2017 ).
Beer is the most popular low-alcohol beverage consumed in huge amounts globally each year. It is important in the economy of many countries around the world (Maicas 2020 ). In Europe, traditional distinctions of beers are influenced by the different brewing practices, the water properties used in brewing, the type of malt and the yeast strains used (Britannica 2022a ). The European countries are some of the major producers and consumers of beers. The primary yeast involved in beer making is S. cerevisiae; however, other species such as S. eubayanus were also recently utilized in the brewing process (Gibson et al. 2017 ). Brewing is a complex fermentation process used in the beverage industries with huge annual revenues (Maicas 2020 ). Global beer production was valued at USD 605.20 billion in 2020 (Allied Market Research 2022b ).
Wine is an alcoholic drink made from fermented grapes. The varieties of grapes are the main factor in wine production. S. cerevisiae is also the main microorganism used in wine making. The global wine market size was USD 339.53 billion in 2020 which is projected to grow to USD 456.76 billion in 2028 (Fortune Business Insight 2022b ).
White spirits
Vodka is the most popular beverage in eastern Europe, traditionally made from potatoes and grain, especially rye (Menezes et al. 2016 ; Britannica 2022b ). The alcohol content of vodka is unaged and high concentration (40%) alcohol by volume (ABV), thus, can cause poisoning if over-consumed (Kanny et al. 2015 ; Pauley and Maskell 2017 ). The vodka industry was worth USD 39 billion in 2020 (Conway 2022 ).
Gin is an alcoholic beverage from junipers that has evolved greatly over the past 300 years from its origin with an alcohol content of 35–55% ABV (Abel 2001 ; Alcohol Rehab Guide 2022 ). It was previously invented as a remedy for military troops suffering from ‘East Indian fevers' (Abel 2001 ). The global gin market was valued at USD 14.03 billion in 2020 , and is expected to reach USD 20.17 billion by 2028 (Allied Market Research 2022c ).
Tequila is a distilled beverage made from the blue agave plant ( Agave tequilana ) originating from the western Mexican state of Jalisco (Graham 2022). It has an alcohol concentration of 38–55% ABV (Alcohol Rehab Guide 2022 ). The size of the tequila market has reached USD 12.89 billion in 2021 (The Spirit Business 2022 ).
Dark spirits
A dark spirit is a type of alcohol that has been aged in oak barrels, resulting in a dark color. With the exception of brandy, which is distilled from wine, dark spirits are beverages distilled from grains.
Rum is a distilled drink made from fermented sugarcane or molasses that originated in the Americas. It has an alcohol concentration of 40% ABV, but can go “overproof” with an alcohol concentration of 57.5–75% ABV (Alcohol Rehab Guide 2022 ). The highest valued rum comes from countries such as Barbados, Cuba, Guyana, Jamaica, and the Philippines (Brick 2022 ). The global value of the rum market was USD 15 billion in 2021, and is expected to reach USD 21.5 billion by 2027 (Market Data Forecast 2022b ).
Whiskey is a spirit made from fermented grains such as barley, corn, rye, and wheat aged in wooden casks. It has a typical alcohol concentration of 40–50% ABV (Alcohol Rehab Guide 2022 ). The global whiskey market was valued at USD 59.63 billion in 2019, and is projected to reach USD 86.39 billion by 2027 (Allied Market Research 2022d ).
Brandy is produced by distilling wine with an alcohol concentration of 35–60% ABV (Alcohol Rehab Guide 2022 ). Brandy varieties are well known throughout the world, such as Cognac and Armagnac from southwestern France (BBC 2022 ). The global brandy market was valued at USD 23.17 billion in 2020 and is expected to reach USD 25.32 billion by the end of 2027 (Globe Newswire 2022a ).
Our economy is significantly impacted by alcohol. Beer and wine were the most popular alcoholic beverages on the market. The total market value for alcoholic beverages made from fungi was USD 1,108.45 billion , demonstrating how popular drinking is worldwide.
Chocolate is primarily produced from cocoa beans ( Theobroma cacao ). Cocoa trees are native to the tropical regions of Central and South America. Most of the flavors that we recognize in chocolate only start to develop during the fermentation of beans (Carvalho 2016 ). Yeasts are also involved in nonalcoholic fermentation of chocolate production to improve chocolate flavor properties and add a new collection of aromas to chocolate (Maicas 2020 ). Yeasts are also responsible to produce several secondary metabolites that are flavor-active that add different fruity aromas to chocolate. These fruity volatile compounds include aldehydes, esters, fatty acids, higher alcohols, organic acids, phenols and sulphur-containing compounds (Carvalho 2016 ). The main yeasts involved in cocoa fermentation are Hanseniaspora opuntiae , Pichia kudriavzevii , and S. cerevisiae , which produce higher alcohols and acetyl-CoA to make acetate esters that give floral and fruity aromas (Gutiérrez-Ríos et al. 2022 ). Other yeasts found in cocoa formation that produce aromas are Galactomyces Galactomyces geotrichus and Wickerhamomyces anomalus (Koné et al. 2016 ). Saccharomyces, Pichia, Hanseniaspora, Kluyveromyces, Hansenula, Wickerhamomyces and Torulaspora are the predominant wild genera of yeast in cocoa fermentation in Colombia (Sandoval-Lozano et al. 2022 ).
There are approximately 4 million exporters of cocoa beans worldwide (International Trade Center 2022 ). The top exporters of chocolate and other food preparations containing cocoa in 2021 with their exported value were Germany (USD 32.82 billion), Belgium (USD 5.37 billion), Italy (USD 2.47 billion), Poland (USD 2.34 billion) and The Netherlands (USD 2.12 billion) (International Trade Center 2022 ). The global chocolate market value reached USD 130.56 billion in 2019 (Grand View Research 2022a ).
Coffee is one of the most consumed nonalcoholic beverages in the world. Due to its popularity and high demand for coffee quality from consumers, constant improvement and increase in variety are deemed necessary (Martinez et al. 2019 ; Ruta and Farcasanu 2021 ). Yeast processing can help to improve the quality and complexity of coffee (Walbank 2022 ). Coffee is an essential commodity exported to around 70 tropical and subtropical countries (FAO 2015 ). The global coffee market was valued at USD 102.02 billion in 2020 (Research and Markets 2022a ). The top coffee exporters according to net traded value were Brazil (USD 5.08 billion), Switzerland (USD 2.71 billion), Germany (USD 2.59 billion), Colombia (USD 2.54 billion), and Vietnam (USD 2.24 billion) (OEC 2022d ). However, like with other goods, it is unclear how much of the world coffee output is dependent on yeast fermentation, so the value provided here is an overestimation, but without more detailed market information, no further breakdowns of this amount can be calculated.
Vinegar is a liquid product formed from alcoholic fermentation and subsequent acetous fermentation of carbohydrate sources. It has been reported as medication in many cultures and documented for its beneficial health effects when consumed regularly (Ho et al. 2017 ) . Vinegar is the product of a two-step fermentation: In the first step, yeast converts sugars from fruits and grains to ethanol anaerobically, while in the second step, ethanol is oxidized to acetic acid aerobically by bacteria of the genera Acetobacter and Gluconobacter . However, alcohol formation is necessary to produce the required percentage of acetic acid (4–5%) for the liquid not to spoil (Tonkinson 2022 ). Several yeast species are involved in the fermentation of vinegar such as in traditional balsamic vinegar including Candida lactis-condensi, C. stellata, Hanseniaspora osmophila, H. valbyensis, S. cerevisiae, Saccharomycodes ludwigii as well as Zygosaccharomyces bailii, Z. bisporus, Z. lentus, Z. mellis, Z. pseudorouxii and Z. rouxii (Solieri and Giudici 2008 ). Vinegar has been an important household commodity and is used around the world. The global vinegar market reached a value of USD 2.27 billion in 2021 (Imarc 2022c ).
Kombucha is a beverage produced from the fermentation of tea ( Camellia sinensis ) by acetic bacteria and osmophilic yeasts (De Filippis et al. 2018 ). It is a good source of probiotics with numerous health benefits. Kombucha is an ancient drink dating back to as early as 220 B.C (Jayabalan et al. 2014 ). The yeasts involved are Brettanomyces/Dekkera , Candida , Torulaspora , Pichia , Schizosaccharomyces , Saccharomyces and Zygosaccharomyces (May et al. 2019 ) . The global market value for kombucha was USD 2.59 billion in 2021 (Polaris Market Research 2021 ).
Organic acids are one of the fastest growing sectors of the fermentation market globally, in which biotechnological production is an imperative economic alternative to chemical synthesis (Sharma et al. 2021 ). Organic acids are extensively used as ingredients in modern food processing (Copetti 2019 ). They are used mostly as buffers, preservatives, flavor enhancers, and adjuvants (Copetti 2019 ). Some organic acids from fungi have already made it to the market, such as citric, gluconic, and lactic acids made from fermentation of glucose or sucrose by Aspergillus niger (Copetti 2019 ). Other organic acids produced mostly by filamentous fungi but used to a lesser extent are fumaric, itaconic, malic, oxalic, succinic, and tartaric acids (Magnuson and Lasure 2004 ; Liaud et al. 2014 ; Dörsam et al. 2017 ).
Citric acid is an α-hydroxylated tricarboxylic acid found in citrus fruits. It has numerous applications in the food, pharmaceutical, and industrial fields. It is used to prevent the crystallization of sucrose in candies, as an acidulant in carbonated drinks, buffering agent, pH stabilizer in fruit and vegetable juices, and antioxidant as preservatives (Grewal and Kalra 1995 ; Magnuson and Lasure 2004 ; Carocho et al. 2018 ). Commercial citric acid production was initially done using A. niger in surface fermentation in 1916 by James Currie (Grewal and Kalra 1995 ; Show et al. 2015 ). Over the years, a variety of other microorganisms were studied, but A. niger is still superior among them in terms of production yield (Show et al. 2015 ). Citric acid production using this filamentous fungus is a multi-billion dollar industry (Cairns et al. 2018 ). Citric acid market was valued at USD 2.81 807 billion in 2021 and reached to USD 2.89 billion in 2022 due to increased demand (Future Market Insights 2022a ).
Fumaric acid is an unsaturated dicarboxyclic acid with various industrial applications in the food industry as a food acidulant in beverages and baking powders, feedstock for the synthesis of polymeric resins, and renewable energy resources (Yang et al. 2011 ). Fumaric acid is produced from glucose fermentation by the filamentous fungus Rhizopus arrhizus via a reductive tricarboxylic acid (TCA) pathway (Pan et al. 2016 ; Martin-Dominguez et al. 2022 ) and had an estimated market value of USD 654.4 million in 2020 (Reportlinker 2022 ).
Gluconic acid was first isolated from a strain of A. niger in 1922 (Goldberg and Rokem 2009 ). The production of gluconic acid can be done on an industrial scale using the enzymes glucose oxidase and catalase, derived from A. niger, which can efficiently convert glucose to gluconic acid (Copetti 2019 ). Other filamentous fungi, such as Gonatobotrys, Gliocladium, Penicillium and Scopulariopsis were found to produce gluconic acid (Goldberg and Rokem 2009 ; Dowdells et al. 2010 ). Gluconic acid and its derivatives have various applications in pharmaceuticals, cosmetics, and food products as additive or buffer salts (National Center for Biotechnology Information 2022b ; Yadav et al. 2022 ). The market size of gluconic acid was more than USD 50 million in 2017(Global Market Insights 2022b ).
Itaconic acid is a dicarboxylic acid with an α,β-unsaturated functionality that can be used as a monomer for the production of resins, plastics, paints, and synthetic fibers (Steiger et al. 2013 ). Aspergillus species such as A. itaconicus and A. terreus have the ability to synthesize itaconic acid (Steiger et al. 2013 ) as well as Ustilago maydis (Wierckx et al. 2020 ). The value of the itaconic acid market was USD 98.4 million in 2021 (Market Data Forecast 2022c ).
Lactic acid is also one of the most common organic acids produced from renewable substrates using bacteria and fungi such as Rhizopus species (Zhang et al. 2007 ). Lactic acid became also interesting due to its high added value (Castillo Martinez et al. 2013 ). It has various applications in the food and food-related industries (Ameen and Caruso 2017 ). Other potential applications are the production of biobased itaconic acid substitutes for petrochemical derivatives, such as biodegradable and biocompatible polylactate polymers (Zhang et al. 2007 ; Teleky and Vodnar 2019 ). Lactic acid market value was estimated to be USD 1.1 billion in 2020 (Markets and Markets 2022a ).
Fungi-based protein also called mycoprotein, is a sustainable protein source produced from single-cell protein (SCP) production technologies that could address the demands of protein worldwide (Nigam and Singh 2014 ). The global demand on vegetarian opportunities is currently rising, so the market share of mycoprotein is bound to increase. Moreover, mycoprotein consumption has been associated with numerous bioactivities such as antioxidant, antidiabetic, reduced cholesterol level, and fungal proteins are a potential source of amino acids that could induce muscle protein synthesis (Derbyshire and Delange 2021 ; Stoffel et al. 2021 ). Formerly, mycoproteins were produced for animal consumption, but the market for human feed is constantly growing (Nigam and Singh 2014 ; Derbyshire 2022 ). The commercially marketed Quorn™ mycoprotein is derived from Fusarium venenatum (Nigam and Singh 2014 ), which remains the most important source. Pleurotus albidus can also be used to produce mycoproteins flour that was used to replace wheat flour to produce cookies (Stoffel et al. 2021 ). The company “Nature Fynd” promotes Fy produced by a strain of Fusarium flavolapis , collected in the Yellowstone National Park, as an alternative producer. Due to the numerous food applications of mycoprotein, it was valued at USD 156.6 million in 2020 (Allied Market Research 2022e ) and is estimated to attain a market worth of USD 397.5 million in 2029 (Globe Newswire 2022b ) (Table 3 ).
Food enhancers/staple food
Soy sauce is the oldest food flavoring ingredient that is eminent, particularly in Asian countries (Hong et al. 2015 ). It is an all-purpose seasoning used in around 100 countries around the world (Ito and Matsuyama 2021 ). Fungi responsible for brewing soy sauce are known as koji molds and belong to the genus Aspergillus (e.g. A. flavus var. oryzae , A. sojae, and A. tamarii ) (Terada et al. 1981 ). Soy sauce is produced by fermenting soybean, wheat, and salt by the action of koji mold, halophilic lactic acid bacteria, and salt-tolerant yeasts such as Candida etchellsii , C. versatilis, and Zygosaccharomyces rouxii (Ito and Matsuyama 2021 ). The market value of soy sauce was around USD 39.5 billion in 2019 (Verified Market Research 2022b ).
Miso is another condiment, a fermented soybean paste made of Aspergillus species that are involved in making soy sauce, specifically A. flavus var. oryzae and A. sojae (Yokotsuka and Sasaki 1998 ; Allwood et al. 2021 ). Other fungi involved in accelerating miso ripening process are Zygosaccharomyces spp. together with the lactic acid bacterium, Pediococcus halophilus (Wilson 1995 ; Batt and Tortorello 2014; Allwood et al. 2021 ). There are several kinds of miso products depending on the type of koji used in fermentation (e.g. rice, barley, soy bean) (Wilson 1995 ; Allwood et al. 2021 ). The market size for miso was valued at USD 67.8 million in 2022 (Future Market Insights 2022b ).
Indonesian tempeh is a mold-facilitated fermented product produced from fermented soy beans mostly consumed as stable food in Indonesia (Dinesh Babu et al. 2009 ), but is widely accepted globally (Ahnan‐Winarno et al. 2021 ). The fungi involved in soybean fermentation for tempeh production are Rhizopus species such as R. microporus (formerly R. oligosporus ), and R. arrhizus (formerly R. oryzae ) (Maheshwari et al. 2021 ; Sjamsuridzal et al. 2021 ). The mycelia of the Rhizopus species hold the soy beans together, thus forming a compact structure like a cake (Gandjar 1999 ; Handoyo and Morita 2006 ). The global tempeh market was valued at USD 4.53 billion in 2021 (The Business Research Company 2022 ).
Food colorants are pigments added to food to enhance quality and add color to the food to make it more presentable and attractive to consumers (Hyde et al. 2019 ). Natural colors are generally regarded safer than synthetic ones and some may even have medicinal benefits. Furthermore, food colorants are also added to preserve food products (Christiana 2016 ). Nevertheless, the compounds should undergo extensive toxicity tests before they can be added to food, as some fungal toxins are also pigments. With the growing demand for natural food colorants globally, filamentous fungi have become important and readily available sources of food coloring (Dufossé et al. 2014 ; Hyde et al. 2019 ). Several genera of filamentous fungi such as Fusarium , Monascus, Penicillium, and Talaromyces have been explored for colorant production (Caro et al. 2017 ; Lagashetti et al. 2019 ). Marine fungi are also potential sources of pigments with various color hues and atypical chemical structures (Dufossé et al. 2014 ). Filamentous fungi can produce an extraordinary range of pigments, including some chemical classes such as carotenoids, melanins, flavins, phenazines, quinones, azaphilones, and violacein or indigo (Dufossé et al. 2014 ; Poorniammal et al. 2021 ). For instance, the strain Penicillium oxalicum var. armeniaca CCM 8242 isolated from soil was able to produce the first commercial red color Arpink red™ pigment (Natural red™) (Caro et al. 2017 ). The high demand for natural food colorants was reflected in its global market value of USD 1.6 billion in 2020 (Mordor Intelligence 2022 ) (Table 4 ).
Astaxanthin is a red–orange carotenoid belonging to the xanthophyll family (Aneesh et al. 2022 ). The natural sources of astaxanthin include bacteria, fungi, algae, crustaceans, and certain fishes. Important fungal astaxanthin producers are the yeasts Phaffia rhodozyma (Pandey et al. 2015 ) and Xanthophyllomyces dendrorhous (Rodríguez-Sáiz et al. 2010 ). The market price of astaxanthin ranges from USD 2500 to USD 7000/kg with the global market value of USD 1.94 billion in 2022 (Grand View Research 2023a ). However, we did not find a way to discriminate between fungal and non-fungal sources during our literature search.
Carotenoids are terpenoid pigments commonly produced by vascular plants, algae, bacteria, and fungi (Avalos and Carmen Limón 2015 ). Various fungi produced various carotenoids. Among these carotenoid-producing fungi are Blakeslea trispora (zygomycete) , Neurospora crassa (sordariomycete) and Xanthophyllomyces dendrorhous (basidiomycetous yeast) (Avalos and Carmen Limón 2015 ; Sandmann 2022 ). The provitamin β-carotene has also been described in Aspergillus giganteus , Penicillium sp., Rhodosporidium sp., Sclerotium rolfsii , Sclerotinia sclerotiorum , and Sporidiobolus pararoseus. Aside from the food market, there are potential applications for carotenoids in the textile industries (Venil et al. 2020 ). The global market size for carotenoids was USD 1.4 billion in 2019 (Fortune Business Insight 2022c ), but once again, the share of fungal products remains obscure.
Monascus pigments are natural food colorants widely used in the food industries worldwide, but especially in China and Japan (Feng et al. 2012 ). They are produced by fungi belonging to the genus Monascus . The six main pigments produced by strains of the genus Monascus are orange (monascorubrine, rubropunctatin), red (monascorubramine, rubropuntantamine), and yellow (monascine, ankaflavin) (Agboyibor et al. 2018 ). Furthermore, Monascus pigments have a variety of biological activities, such as antimutagenic, anticancer properties, antimicrobial activities, and potential anti-obesity activities. Monascus pigments already had a market value of USD 1 million in Japan at the end of the 1990s (Dufossé 2019 ), but we did not find hard data on the current market (Fig. 6 ).
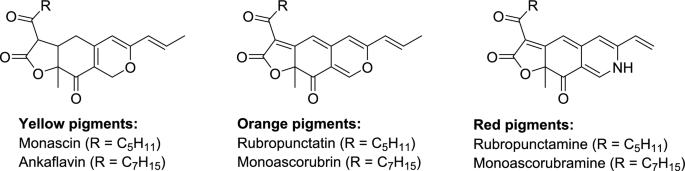
Chemical structures of Monascus pigments
Another application of natural dyes from fungi is the textile industry (Chadni et al. 2017 ). Some wood rotting fungi are utilized in the production of textile dyes (Hernández et al. 2019 ). Other filamentous fungi can also produce various pigments with application in dyeing cotton, silk, and wool (Sudha et al. 2016; Kalra et al. 2020 ). Neurospora spp. are ascomycetous yeasts that can also be a great source of pigments (Lagashetti et al. 2019 ; Kalra et al. 2020 ).
Agarwood is a dark resinous heartwood that forms in mainly Aquilaria and Gyrinops trees naturally infected with endophytic fungi such as Cladosporium, Fusarium, Melanospora spp ., and “Mycelia sterilia” (Hyde et al. 2019 ), however, fungi reported have not been identified accurately according to the current standards. Agarwood is used as a fragrance in cosmetics and some important religious rituals (Chowdhury et al. 2016 ). The global market value of the agarwood chip market is worth USD 8.30 billion in 2018 (Straits Research 2021 ). Agarwood oil also has applications in the chemical, cosmetics, and personal care product industries with a global market value of USD 278.03 million in 2021 (Maximize Market Research 2021 ) (Tables 6 , 7 ).
Fungi synthesize natural active biomolecules that can be applied to develop cosmetic products due to their important biological functions and applications such as antiaging, antioxidants, skin revitalization, skin whitening, and hair products (Hyde et al. 2010 ; Wu et al. 2016 ; Badalyan et al. 2022 ). Numerous bioactive metabolites such as polysaccharides and glycoproteins, as well as other metabolites such as phenolic compounds, terpenoids, and several lipid components, have been explored due to their functions in skin health (Usman et al. 2021 ).
Lentinula edodes and Ganoderma species are two of the most popular fungal sources used in skin care products. Lentinan extracted from L. edodes has the potential to protect the skin against environmental pollutants (Zi et al. 2020 ). Agaricus subrufescens residues were also used as cosmeceutical ingredients (Hyde et al. 2010 ; Wisitrassameewong et al. 2012 ). Other macrofungal species utilized in skin care preparations include Taiwanofungus camphoratus, Grifola frondosa, Inonotus obliquus, Ophiocordyceps sinensis , and Schizophyllum commune (Wu et al. 2016 ). The following compounds are economically important isolated from fungi.
β-Glucans are polysaccharides that constitute around 30–80% of the fungal cell wall (Free 2013 ). Among these β-glucans, lentinan had a market value of USD 10 million in 2022 (Market Watch 2023b ). However, yeast is the main source of β-glucans in the market with a global value of USD 174.2 million in 2021 (Grand View Research 2023b ). The global β-glucan market was over USD 329.4 million in 2019 (Global Market Insights 2022c ) and increased to USD 403.8 million in 2020 (Markets and Markets 2022b ).
Ergothioneine is a natural sulphur derivative of histidine, which was originally isolated from the ascomycete Claviceps purpurea (Tanret 1909 ). It has antioxidant activity and is nowadays more commonly produced from edible mushrooms (Fu and Shen 2022 ). Some sources of ergothioneine are A. bisporus, Boletus edulis Flammulina “ velutipes ” (probably F. filiformis according to the current taxonomy established by Wang et al. 2018), Pleurotus eryngii, P. ostreatus and Lentinula edodes (Lee et al. 2009 ; Kalač 2016 ). The global market value of l -ergothioneine was around USD 15.02 million in 2021 (Market Data Forecast 2022d ).
Kojic acid , a pyrone derivative, is a byproduct in the fermentation process of producing sake, an alcoholic drink made from rice and almost exclusively produced in Japan (Goldberg and Rokem 2009 ). It is a metabolite of Aspergillus species, including A. oryzae and A. flavus. Kojic acid inhibits tyrosinase in the synthesis of melanin and is documented for its numerous bioactivities (Saeedi et al. 2019 ). It is known for its radioprotective and skin lightening effect and therefore has been used as ingredients in the production of skin creams, lotions, soaps and dental care products (Saeedi et al. 2019 ; Phasha et al. 2022 ). Kojic acid has a global market value of USD 36 million in 2022 (Market Watch 2022c ).
Fungi have been used as biological control agents of crop pests in agricultural and urban areas for several decades. (Wraight and Carruthers 1998). Almost 50% of the registered microbial biopesticides are based on fungi (Mascarin et al. 2018). Mycopesticides have been on the market since 1981, and the first of these products was registered in the United States as Mycar. It was composed of Hirsutella thompsonii , which causes epizootics in some species of spider mites (Rechcigl and Rechcigl 2000). Mycoinsecticides are interesting organic alternatives to chemical insecticides because they tend to be less harmful to human health and the environment. They are also target-specific with less detrimental effects to human and pets (Moore et al. 2011).
The most explored entomopathogenic fungal genera are Akanthomyces, Beauveria, and Metarhizium, which can infect a wide variety of insect hosts such as dipterans, lepidopterans, and coccidae. Numerous studies have explored the efficacy of these genera as biocontrol agents. Beauveria bassiana is one of the most explored entomopathogenic species worldwide (Fancelli et al. 2013 ). It has high genetic variability, virulence and adaptability to local conditions (Fancelli et al. 2013 ). Metarhizium, on the other hand, has been widely studied because of its general safety, narrow host range, environmentally friendliness and ease of mass production (Aw and Hue 2017). The global biopesticides market is estimated to be valued at $ 5.5 billion in 2022 (Research and Markets 2022b ). Mycopesticides account for around 10% of the global market value of biopesticides (Zaki et al. 2020 ), thus the global market value of mycopesticides can be estimated to be approximately USD 550 million in 2022.
Strobilurins are economically important bioactive compounds first reported by Anke et al. ( 1977 ) from cultures of the basidiomycete Strobilurus tenacellus. These compounds have later also been extracted from numerous Basidiomycota such as Oudemamsiella canari and Mucidula mucida (Rosa et al. 2003 , 2005 ; Iqbal et al. 2018 ). Strobilurins became an inspiration in developing the β-methoxyacrylate class of agricultural fungicides (Nofiani et al. 2018 ) such as azoxystrobin (Quadris), trifloxystrobin (Flint), pyraclostrobin (Cabrio), or Pristine (pyraclostrobin and boscalid, a premix of a Group 11 and a Group 7 fungicide). The global market value for strobilurin fungicides was estimated to be USD 4.65 billion in 2022 (Market Watch 2022d ).
Mycorrhizal fungi such as arbuscular mycorrhiza and ectomycorrhiza are considered natural biofertilizers since they provide important nutrients to the plant in exchange for photosynthetic products (Berruti et al. 2016 ; Domínguez-Núñez et al. 2020 ). A mycorrhiza-based biofertilizer is a substrate containing fungi colonizing the rhizosphere or the interior of the plant when applied to seeds, plant surfaces, or soil (Hyde et al. 2019 ; Karima and Samia 2020 ). It helps in plant growth by enhancing the supply of primary nutrients to the host plant and promoting growth hormones (Berruti et al. 2016 ).
The most widespread EcM product inoculum is Pisolithus tinctorius with a wide host range that can be applied as a vegetative mycelium peat vermiculite carrier used in nursery and forestry plantations (Gentili and Jumpponen 2006 ; Sebastiana et al. 2018 ). Other fungi used are Rhizophagus (formerly Glomus ), Sebacinales and Trichoderma species (Kaewchai et al. 2009 ; Molla et al. 2012 ). The market value of mycorrhiza-based biofertilizers was USD 271.8 million in 2021 (Market Data Forecast 2022e ).
Fungi grow ubiquitously on complex organic matter, prone to recycle nutrients and decompose complex substrates. In order to successfully accomplish this endeavor, they are equipped with a great portfolio of enzymes. Compared to chemical reactions, enzymes, used as biocatalysts, are highly specific, have few side products, and show mild reaction conditions with a low environmental and toxicological impact. Besides highly specific ones, also enzymes with a broad substrate spectrum are present. In general enzymes are classified in six classes by the International Enzyme Commission: EC1 oxidoreductases, EC2 transferases, EC3 hydrolases, EC4 lyases, EC5 isomerases, and EC6 ligases. In 2019 fungal enzymes had an overall marked share of 50% on all enzymes available (Kango et al. 2019 ). Despite of the large share, solely few species, namely Aspergillus , Trichoderma , Rhizopus , and Penicillium, contribute significantly to the market (El-Gendi et al. 2021 ).
In the food processing industry, fungi are the primary source for enzymes. They are applied to influence for example food quality, shelf life, texture, color, aroma but may also simplify production steps and yields (Zhang et al. 2018 ). Due to their unique properties, they are also used in the pharmaceutical, chemical, textile, detergent, cosmetic, and flavor or fragrance industry (He et al. 2017 ; Haile and Ayele 2022 ; El-Gendi et al. 2021 ). Whereas in 1983 about 30 enzyme classes, half of fungal origin, were commercialized, in 2020, the industrial enzymes market was valued at USD 5.7 billion (PS Market Research 2022 ). Though no detailed information on product origins is available, several global acting companies for enzyme production use fungi as production organisms. Among them are Novozymes (Denmark), DSM (Netherlands), Chr. Hansen (Denmark), DuPont (USA), BASF (Germany), Advanced Enzymes (India), and AB Enzymes (Germany). Here, production processes are diverse. Besides cultivation of the native producer strain, also heterologous hosts are widely spread. Second helps to overcome to long cultivation times, process in homogenities and simplifies the overall process. However, in case of technical enzymes, partially purified enzyme mixtures decrease production costs significantly. In bioremediation, wastewater treatment and bioethanol production, the use of whole-cell bioconversion of non engineered strains in mixed cultures is preferred to enzyme preparations (El-Gendi et al. 2021 ). Continuous fermentation simplifies processes and keeps them at low costs. Modern bioinformatics pushes the identification of novel enzymes with unique properties. Furthermore, genome mining approaches are on the verge, which increases product portfolios drastically.
Oxidoreductases are catalyzing redox reactions, therefore are typical housekeeping enzymes i.e., involved in the glycolysis and amino acid metabolism. Despite the great potential and the formation of several products of industrial interest, oxidoreductases are nowadays solely used in niche applications (Gygli and van Berkel 2015 ). However, fungi have a large share of this market, especially in bioremediation applications and wastewater treatment (Verma et al. 2020 ). This is due to the fact that mainly white rot Basidiomycota grow on lignocellulolytic biomass hitherto secretes robust enzymes of high redox-potential for the substrate degradation (Martínez et al. 2018 ). Especially Laccases, monooxygenases, and dioxygenases are of commercial use (El-Gendi et al. 2021 ).
Laccases have a great potential to oxidize phenolic and non-phenolic substrates cofactor independently. They are commercially used in destaining processes, paper industry, medical applications, and for biosensors. Usually, fungi are applied in microbial consortia to degrade pollution i.e. Aspergillus consortia that are used in wastewater treatment to degrade heavy metals chromium and cadmium (Talukdar et al. 2020 ). The global market value for laccase was USD 3 million in 2021 (Business Research Insights 2022 ).
Reactions involving the transfer of functional groups are catalyzed by transferases. Especially enzymes, catalyzing the transformation of higher sugars, are of commercial use. Invertases are breaking down sucrose into fructose and glucose (Nadaroglu and Polat 2022 ). Fungi that produce invertases are Aspergillus, Penicillium , Rhizopus and yeasts such as Saccharomyces (Kulshrestha et al. 2013 ; Matei et al. 2017 ) . Invertase is prevalent in the food industry, especially in making confectioneries and baking as well as extending the shelf life of the food products (Infita 2022 ). Inverted sugar prevents crystallization in food products and increases sweetness of soft fillings like soft caramel (Verma et al. 2020 ). Moreover, fructosyltransferase activities are used to produce fructooligosaccharides (FOS) which are known for their health promoting effects. FOS are applied as an alternative sweetener with a viscous texture in food industry (Lateef et al. 2012 ). The overall invertase market was valued at USD 57.6 million in 2022 (Future Market Insights 2022c ).
Hydrolases can cleave substrates via the addition of water. They display the most extensively studied enzyme class with the largest potential for commercialization (Gurung et al. 2013 ). Especially fungal amylases, proteases, cellulases and lipases are widely commercialized, but also other enzymes of industrial interest are known.
Among them, α -amylases are randomly hydrolyzing α-1,4-glycosidic bonds converting complex carbohydrate to simple sugars (Akinfemiwa et al. 2022 ). This unique property allows cleavage of non-reduced sugar residues unlike other amylolytic enzymes (Gupta et al. 2003 ). α - Amylases have the largest share of enzyme preparations used in industry (Monteiro de Souza and De Oliveira e Magalhães 2010 ). The global market size was valued at USD 278.2 million in 2018 and expected to increase up to USD 353 million by 2026 (Fior Market 2022 ). Among others, they are applied in the food, detergent, pulp and paper, and textile industry to hydrolyze starch (Gupta et al. 2003 ). The main applications of α -amylases are in the production of bakery products and food processing i.e. for glucose production (Copetti 2019 ). Herein, fungal enzymes are preferred to other sources due to their GRAS-status (Gupta et al. 2003 ). In bakeries, they are applied since 1995 and lead to a higher dough volume, better color, softer doughs, higher toasting quality, and a softer crumb (Pritchard 1992 ). They are commercially produced by Aspergillus (e.g. A. awamori , A. niger and A. oryzae ), Penicillium and Rhizopus (Pandey et al. 2000 ; Copetti 2019 ).
Proteases hydrolyze peptide bonds of proteins into smaller polypeptides or single amino acids (Flores-Gallegos et al. 2019 ). Various fungi such as Aspergillus, Humicola, Mucor, Penicillium, Rhizopus, Thermoascus, Thermomyces , etc. can produce proteases (Monteiro de Souza et al. 2015 ). These enzymes are easier to purify than their bacterial counterparts (Vishwanatha et al. 2010 ). Proteases have various industrial applications in the food industry, as pharmaceuticals and in biomedical therapies (Monteiro de Souza et al. 2015 ; Agbowuro et al. 2018 ; Naeem et al. 2022 ). Fungal proteases are cost effective and have faster production compared to other microbial sources (Monteiro de Souza et al. 2015 ). The global market value of fungal proteases was valued at USD 1.3 billion in 2021 (Dataintelo 2022a ).
Increasing demand on biofuels opens the potential of cellulose as cost effective substrate. Degradation is catalyzed by cellulases . Apart from biofuels, cellulases are also applied in combination with pectinases to clarify juices and in biopolishing of textiles (Gurung et al. 2013 ). Because of its high product titers, exceeding 100 g/L in submerged processes, Trichoderma reesei is widely used in industry (Gupta et al. 2016 ). The global market value of cellulases was USD 1.62 billion in 2022 (Future Market Insights 2022d ).
Pectinases are catalyzing the hydrolysis of pectin and pectic substances. Pectinases have wide application in food industry especially in fruit juice extraction to increase pressing efficiency and to clarify the former turbid drink (Jayani et al. 2005 ). Enzyme treatment in combination with cellulases decreases filtration times by 50% (Blanco 1999 ). During textile production an enzymatic cocktail containing among others pectinases is applied to remove non-cellulolytic impurities from clothes, called biosourcing (Hoondal et al. 2002 ). Some of the most efficient fungal species in producing pectinases are Aspergillus awamori, A. niger, Mucor piriformis, Penicillium restrictum, Trichoderma viride, and Yarrowia lipolytica (Haile and Ayele 2022 ). About 25% of global share of food enzyme in 2005 sales pectinases with the market value of USD 1.4 billion in 2020 based on the global industrial market value of industrial enzymes (Jayani et al. 2005 ; PS Market Research 2022 ).
Lipases hydrolyze triacylglycerols to glycerol and fatty acids which is of commercial interest as biological laundry detergents, cosmetics additive, fine chemical production, paper pitching, leather defatting, wastewater treatment, and biodiesel production (El-Gendi et al. 2021 ). Fungal lipases stand out due to its alkaline and temperature stability (Singh and Mukhopadhyay 2012 ). In food production i.e. offered by Rohm and Haas Co. USA, lipases are used to improve cheese aroma (Singh and Mukhopadhyay 2012 ). Important sources for fungal lipases are for example Aspergillus , Penicillium , and Fusarium (El-Gendi et al. 2021 ). The global lipase market value in 2022 was USD 694 million (Future Market Insights 2022e ).
Galactosidases , specifically β -galactosidases isolated from bacteria, filamentous fungi and yeasts, are responsible to hydrolyze lactose (Saqib et al. 2017 ). Yeasts, such as Kluyveromyces lactis and Guehomyces pullulans , but also filamentous fungi like Aspergillus niger are commercially important sources of β -galactosidases for food processing, waste disposal, and prebiotic production (Hu et al. 2010 ; Saqib et al. 2017 ). This enzyme is also used in whey disposal, a byproduct of cheese industry (Karasova et al. 2002 ). Enzymatically treated waste streams are rich in ethanol and a sweet syrup that is used further in food production including bakery (Zhou and Chen 2001 ). Lactase is a β -galactosidase, with a special importance for the production of lactose-free milk and dairy products (Copetti 2019 ). This enzyme is industrially obtained from fungal sources such as Aspergillus , Kluyveromyces, Trichoderma, Penicillium, Rhizopus, and Fusarium (Mahoney 1985 ; Zhou and Chen 2001 ; Seyis and Aksoz 2004 ; Jesus and Guimarães 2021 ).. The lactases produced by filamentous fungi are more heat-stable compared with the ones originating yeasts (Copetti 2019 ). Whereas the global market of galactosidases was valued at USD 1.5 billion in 2021, lactase take about 12% of the overall market share with around USD 185.2 million in 2019 (Grand View Research 2022b ; Dataintelo 2022b ).
Lyases mediate elimination reactions. Among them is the pectin lyase of industrial interest, catalyzing the non-hydrolytic cleavage of pectin. As previously described for pectinases, the lyases are present in preparations for fruit and juice processing (Suhaimi et al. 2021 ). These enzymes are also applied in a cocktail with various hydrolases in wine making as well as paper production processes. As for pectinases fungal sources play an important role in production due to their unique enzyme properties, namely temperature and pH stability. Prominent sources are Aspergillus , Candida , Penicillium , Trichoderma , and some yeasts.
Isomerases catalyze rearrangement processes. The most notable is the isomerization of glucose to generate high fructose corn syrup (HFCS), which has various applications in the food sector owing to its sweetness. HFCS has a high solubility, low crystallization tendency, and a high freezing point depression, which makes its use in drinks and frozen desserts advantageous. Besides food, HFCS is used in the detergent, and pharmaceutical industries (Singh et al. 2017 ). Commercially available pectin lyases have been solely reported from Aspergillus .
Ligases catalyze the formation of new bonds. Despite the high importance in intercellular mechanisms (besides niche applications in diagnostics, and molecular biology), hardly any commercialization is reported. Solely yeasts were reported as promising producer strains.
Edible mushrooms
Wild and commercial fungi can be resources of low-calorie functional food and nutraceuticals (Hyde et al. 2019 ; Lu et al. 2020 ). The popularity among consumers is due to the pleasant and unique flavors and health benefits of these mushrooms (Barros et al. 2008 ; Ache et al. 2021 ). Fungi are rich in crude fibers, proteins, vitamins, and minerals; they contain low fat, calories and high quality carbohydrates (Bandara et al. 2017 ; Thakur 2020 ). Fresh fungi have a moisture content of about 90% while dry matter consists of carbohydrates (50–65%), proteins (19–35%), essential fatty acids (2–6%) with some vitamins and minerals (Rathore et al. 2017 ; Jacinto-Azevedo et al. 2021 ). Consumption of edible fungi promotes good health caused by synergistic effects of the bioactive compounds present such as β-glucans, ascorbic acid, lectins, unsaturated fatty acids, phenolic compounds, tocopherols, and carotenoids (Varghese et al. 2019 ). Cultivated (54%), medicinal (38%), and wild fungi (8%) are the three major components of the global mushroom industry (Royse et al. 2017 ).
The world production of mushrooms was 42,590 thousand tons from the 20 largest producing countries, mainly contributed by China (93.93%) in 2020 (Table 8 ) (FAOSTAT 2022a ). The mushroom and truffle production and yield in Asia was around 7 million tons and 3.2 million hg/ha yield, respectively, in 2018. China is the largest contributor with 6.7 million tons production (FAOSTAT 2022a ). The total value for each country was determined by multiplying the production by the 2020 mushroom price (USD/ton) (FAOSTAT 2022b ). The total value of mushroom was USD 45.42 billion in 2020. The value of mushrooms being traded for food which only include cultivable and wild edible fungi constitute to 62% of the mushroom production or USD 28.16 billion in 2020.
Cultivable fungi
Cultivated edible mushroom production is attributed to 54% of global production, amounting to USD 34 billion in 2013 (Royse et al. 2017 ). Around 60 species of fungi are commercially cultivated (Chang and Miles 2004a ). The most cultivated mushroom genera in global production are Agaricus bisporus , followed by “ Lentinus” (Lentinula) edodes , Pleurotus spp., and Flammulina “velutipes” (or F. filiformis ) (cf. Valverde et al. 2015 with the names corrected to the currently valid taxonomy). These genera accounted for 85% of global mushroom production in the world (Royse et al. 2017 ; Kant Raut 2019 ) . The market value of edible cultivated fungi in 2020 accounted to 54% of global production was USD 24.53 billion .
Wild edible fungi
Approximately 2000 species of wild fungi are edible and can be consumed safely (Li et al. 2021 ). About 470 species have medicinal values, and another 180 species have been attributed value in other activities, such as religious purposes. In Tanzania, for instance, macrofungal collectors were able to collect up to 1500 kg of fruiting bodies of wild fungi that can earn around USD 500 to 600 per season (Tibuhwa 2013 ; Chelala et al. 2014 ). The retailers were also able to sell 750 to 800 kg with seasonal earnings of USD 750 to 1000 (Chelala et al. 2014 ). Wild edible fungi are collected each year in several million tons, accounting for 8% of global macrofungal production (Royse et al. 2017 ), therefore it was accounted to around USD 3.63 billion in 2020 .
Facing the transition towards a sustainable economy based on reduction of non-renewable materials and minimization of waste, fungal biotechnology offers a fascinating opportunity with high innovation potential (Meyer et al. 2020 ). In the last two decades, fungal biomaterials attracted growing attention in academic and commercial fields. Transferring organic agricultural or industrial waste into new bio-based products, they constitute a new form of a cost-effective and low energy bio-fabrication (Jones et al. 2020 ). Fungal mycelium has the capacity to degrade lignocellulosic materials and form composite networks (Sun et al. 2019 ) with insulating, non-flammable or hydrophobic properties and an improved mechanical strength (Jones et al. 2020 ). The mycelium binder constituent interfaces a dispersed phase of agricultural residue (substrate filler) and functions as a load transfer medium between the typically fibrous agricultural residue within the composite in a manner similar to the matrix phase of a polymer composite (Jones et al. 2020 ). Produced as an eco-friendly alternative to petroleum-based products, mycelium-based materials are considered to play a pivotal role in construction, automotive, transportation, electronic, and fashion sectors as well as design or applied arts. They comprise a great opportunity for substitution of traditional materials, such as timber, steel, concrete, foams or leather might (Karana et al. 2018 ; Attias et al. 2020 ; Silverman et al. 2020 ; Stelzer et al. 2021 ). Across the diverse disciplines, potential applications for mycelium-based products are reaching from packaging materials, bricks, acoustic panels, insulation panels, wall panels, fireproof material to leather and clothes.
Consistent with an increasing number of patent documents and scientific publications since 2007, the field of mycelium-based materials is currently under rapid development (Cerimi et al. 2019 ; Sydor et al. 2021 ). Sydor et al. ( 2021 ) identified 153 patent applications and 55 patents granted until April 2021, demonstrating the immense attraction for commercialization. US-based companies, such as Ecovative (Ecovative Design LLC 2023 ) and MycoWorks (MycoWorks 2023 ), pioneer companies focusing on dehydrated mycelium materials for packaging, insulation, fire protection or the production of a fungal leather alternative, respectively, have become key players on the market during the last fifteen years. Another up-coming label based in the US and Netherlands is Bolt Threads. In 2018, nine years after their foundation, they have developed the sustainable leather-like material Mylo™ attracting the attention of serious partners like Adidas, Kering, Lululemon or Stella McCartney. Furthermore, the success of European startups indicates the surging demand on the market for economical and eco-friendly sustainable technologies (Jones et al. 2020 ; Bitting et al. 2022 ). Striking examples are MycoTex (NEFFA 2023 ), the winner in 2018 of the Global Change Award created by the H&M foundation, or Grown.Bio (GrownBio 2022 ), featured as the most innovative SME (small and medium-sized enterprise) of the Netherlands in 2021.
Industries like packaging, plastic, and fashion have shown great interest in eco-friendly products (Research and Markets 2022c ). This shift goes hand in hand with an increasing economic impact of mycelium-based products. According to market analyses, the global market for mycelium materials, including food and beverage products, is valued at USD 2.48 billion in 2020 (Research and Markets 2022c ). A year later, the global mycelium market has increased to USD 2.95 billion with North America having the highest share of the mycelium market in 2021, followed by Europe and the Asia Pacific (InsightAce Analytic 2021 ). Driven by an ongoing trend to a bio-based circular economy around the world, governmental regulations, such as the styrofoam and polystyrene bans in various nations, increasing R&D investments and further application opportunities (e.g. mycorrhizal fungal concentrate for organic farming and tree nurseries, health-conscious products), the demand for eco-friendly mycelium-based products is continuously increasing. In 2030, the mycelium market is expected to reach USD 5.49 billion, with a CAGR of 7.3% during a forecasted period of 2022–2030 (InsightAce Analytic 2021 ). One of the key factors in hampering the market growth is the conversion from a small-scale of commercial products into an industrial-size scale. Limiting factors can be depicted by the lack of infrastructure facilities and challenging scale-up processes. Applicable solutions are needed for deficiencies in scalability (e.g. long cycles of production, risk of contamination, complex multi-step manufacturing processes), reproducibility (e.g. missing levels of standardization), and automation (InsightAce Analytic 2021 ; Bitting et al. 2022 ). The monopoly of mycelium-related patents in the industry together with a disconnect between industry and academia is further strengthenig this dilemma and prevent the distribution of knowledge and progress (Bitting et al. 2022 ).
Recreational mushroom picking is another service provided by forest ecosystems in which people can also sell the fungi they collected at the local market (Martínez de Aragón et al. 2011 ; Cutler II et al. 2021 ). Mushroom pickers benefited both from the recreational experience and the product collected (Martínez de Aragón et al. 2011 ; Debnath et al. 2019 ). Public demand for recreational mushroom picking has increased in the past decades in the forest ecosystems, affecting forest land owners (Frutos et al. 2009 ). Many people pick mushrooms for recreational purposes and not for economic reasons; thus, environmental valuation methods allow the benefits of this recreation activity to be assessed. The most common method used to measure the monetary value of recreational mushroom picking is the travel cost method. This method is used to approximate the difference between the willingness to pay for the good of the consumer (demand function) and the actual spending (price) (Bockstael 2007 ).
Mushroom picking is an upward leisure activity in European forests (Schulp et al. 2014 ; Marini Govigli et al. 2019 ). Along with the economic growth of European countries, fungal picking has transformed its profile to that of a primarily recreational activity (Kotowski 2016 ). Most studies of mushroom picking activities are done in Spain (Table 4 ). It shows that Europeans enjoy mushroom foraging activities as leisure activities. For instance, in Spain many studies were conducted in forested areas using the travel cost method to estimate the amount paid for mushroom hunting, which ranged from 10 to 60 €/trip (10.5–63 USD/trip) (Frutos et al. 2009 ). The estimated recreational surplus in Catalonia, Spain was €39 (40.9 USD) per journey (Martínez de Aragón et al. 2011 ). The average recreational surplus in Spain is USD 33.0 per trip, with 23% of the adult population experiencing mushroom picking at least once a year (Martínez de Aragón et al. 2011 ). Spain has around 38.6 million adults (World Population Review 2022 ), which, multiplied to 0.23 is equal to 8.9 million adults, which are experiencing mushroom picking once a year. Each of these 8.9 million adults has a recreational surplus of USD 33.0 per trip, thus the total recreational surplus is USD 293 million per year in Spain.
With filamentous fungi and yeasts being veritable cellular factories, they are major contributors to biotechnology, providing virtuous outlets for the protection of the environment and health by breaking down various industrial waste.
The spent mushroom substrate (SMS) is composed of fungal mycelia, extracellular enzymes, and unused lignocellulosic substrates. Production of industrially important hydrolytic enzymes by fungi has various economic applications, which can be an inexpensive alternative energy source (Grujić et al. 2015 ). SMS is suitable in a fermentative process with easy and low-energy sugar conversion through various hydrolysis methods, such as that leading to the production of economically important bioethanol (Antunes et al. 2020 ). SMS use could be a strategy to improve yield and reduce the cost of the production process (Corrêa et al. 2016 ). It could also be incorporated as a substrate for biogas generation (Pérez-Chávez et al. 2019 ).
Biofuels produced from renewable lignocellulosic biomass are projected to meet increasing energy demands without increasing greenhouse gas emissions as do fossil fuels (Saini and Sharma 2021 ), with butanol as the most promising for its high energy density (Cascone 2008 ). The spent mushroom substrate was also used as a potential substrate for butanol production using biodiesel removal in situ (Antunes et al. 2020 ). The old macrofungal-growing substrates harbor bacteria that can convert cellulose to biobutanol. Biobutanol is an alternative fuel that can directly replace gasoline in engines (Zhen et al. 2020 ). However, traditional methods in producing biofuels require a lot of energy and water. They are costly and require complicated pretreatments (Saini and Sharma 2021 ).
The global biofuels market was valued at nearly USD 110 billion in 2021 and is projected to continue to increase until 2030 with a forecast value of USD 201.2 billion (Statista 2022a ).
Environmental pollutions, especially synthetic organic compounds such as xenobiotics, have become a major problem worldwide. These compounds are recalcitrant and many microorganisms cannot degrade them (Embrandiri et al. 2016 ). Fungi can act as remediation tools due to their ability to enzymatically convert different type of pollutants into less complex components, even including a wide range of xenobiotics (Kulshreshtha et al. 2014 ; Zhen et al. 2022 ). White-rot fungi are able to produce extracellular ligninolytic enzymes consisting of three groups: lignin peroxidase (LiP), manganese-dependent peroxidases (MnP) and laccases, which play important roles in the transformation and mineralization of organic contaminants (Wang et al. 2009 ; Bulkan et al. 2020 ).
In agriculture, contaminated soil with heavy metals has negative effects on crop quality and yield (Kumar et al. 2018). There are three general effective strategies involving fungi to address contaminated soil: biodegradation, bioconversion, and biosorption. Toxicity reduction by fungi depends on their ability to produce different enzymes to degrade pollutants. Fungi can degrade pollutants and transform them into simpler mineral constituents (Chugh et al. 2022 ). They can also convert waste and other pollutants into more useful forms. Fungi can also absorb heavy metals from substrates without production of secondary pollution produced, which could be a very effective method to reclaim polluted land (Prenafeta-Boldú et al. 2019 ). Fungi have the potential to accumulate heavy metals in their network of hyphae and convert them into their mineral constituents. Fungi can also degrade recalcitrant hydrocarbon contaminants. They can turn hydrocarbon pollutants present in the environment into an energy source, increasing the selection of the hydrocarbon-metabolizing fungal population (Prenafeta-Boldú et al. 2019 ). Some fungal species are effective degraders of high molecular weight polycyclic aromatic hydrocarbons (Al Farraj et al. 2020 ). Some genera of fungi used for degradation of polycyclic aromatic hydrocarbons and polycyclic aromatic hydrocarbons are Agaricus (Li et al. 2010 ), Armillaria (Hadibarata and Kristanti 2013 ), Ganoderma (Agrawal et al. 2018 ), Marasmiellus (Vieira et al. 2018 ), Phanerochaete (Wang et al. 2009 ), Pleurotus (Li et al. 2010 ; Hadibarata and Teh 2014 ), and Trametes (as Coriolus ) (Jang et al. 2009 ). However, these projects are all at experimental stages. Among the different potential fungi, only Phanerochaete chrysosporium has been explored in large-scale biodegradation programs. Although global bioremediation has a large market value of USD 12.38 billion in 2021 (Grand View Research 2023c ), contribution of fungi is yet to be determined.
Fungi have been utilized in different industries (Hyde et al. 2019 ). Fungal products and services have a great impact in the global economy. High-value products biosynthesized by fungi can generate millions of dollars, which can help the country’s economy. The valuation of fungi at the global level is exceptionally difficult, and, in this account, we do not claim to accurately estimate this. However, we have used our knowledge and the available literature to derive a starting figure that can be used and built upon openly. Without such a figure, the value of fungi will not be recognized and there will be no monetary value for these amazing organisms, extrapolate decisions from stakeholders to further protect and conserve the mycobiota. This is our first attempt to give monetary value to the contributions of fungi in global economy that focus on marketed products and services. Our evaluation is carried out to derive an internationally accepted figure. The most significant fungal products and services with great economic value are listed in Table 9 . Fungi can be primary source in making products such as medicines and pharmaceuticals, TCM, functional food and nutraceuticals, pigments, and others or they can be involved in the production process especially in fermentation. For instance, production of fermented food and beverages such as baked goods, cheese, alcoholic beverages (e.g. beer, wine, whiskey) and non-alcoholic beverages (e.g. chocolate and coffee) with high market value will not be possible without the activity of yeast and filamentous fungi in fermentation in combination with bacteria. Therefore, involvement of fungi in this important process is also considered as contribution of fungi to global economy.
Our attempt to give monetary value to the contributions of fungi includes the definite value of fungi itself (e.g. wild and cultivated mushrooms), product value from the products made from fungi or through the involvement in the production process, and traded value from fungal services such as carbon stock and recreational foraging. Table 10 is the summation of the market values of fungal products and services that are economically of great importance. The contribution of fungi to the global market through fungal products and services is estimated to be USD 54.59 trillion .
The traded value of fungal services accounted for 95.90% of the total estimated value of fungi, or USD 52.33 trillion (Table 11 ). However, because this is just a monetary estimate of services rendered by fungi and has not been in the actual market, it can only be considered potential market value and not actual market value that affect the global Gross Domestic Product (GDP). The actual market value is made up of definite and product values that are already considered market revenue, totaling USD 2.24 trillion . The GDP is USD 88.44 trillion (Statista 2022b ), thus fungal products constitute for 2.53% of the GDP .
Fungi are being exploited in the forest and other ecosystems for their food production, medicinal and pharmaceutical applications; hence, they represent a major economic source worldwide (Zotti et al. 2013 ). Fungal products are sustainable, which could also create a biobased circular economy, which aims to eliminate waste and transform the production and use of goods (Valavanidis 2018 ). The circular economy is estimated to offer up to USD 4.5 trillion in global economic benefits by 2030 (World Economic Forum 2022 ).
Some services offered by fungi are eminent, especially those with direct effect to humans such as food source, medicines, commodities, and cultural services. These services also have available market values and thus are quantifiable. However, ecosystem services provided by fungi and their role in ecosystem functions, are not recognized, especially those which belong to supporting and regulating services. These services are not generally considered within policy appraisal at present. They represent an area where a greater and more methodical focus would be very valuable. To recognize and improve their strategic importance, several conservation strategies are necessary (Zotti et al. 2013 ). Institutional environment moves towards regulating mycological resources, with estimating the value of this ecosystem service becoming a key tool for policy-makers and rural entrepreneurs (Marini Govigli et al. 2019 ).
A formal ecosystem assessment could provide the necessary information on the larger suite of ecosystem services contributed by fungi in the ecosystems and for beneficiaries to value these services. Smaller and more specific studies should also be performed for each service to capture the true values of fungi. The under-representation of fungal ecosystem services and functions in ecosystem assessments potentially limits the information available for decision-making about regional and global activities that impact forest ecosystems. The concept of ecosystem services was developed as part of the Millennium Ecosystem Assessment project in the early 2000s to analyze and quantify the true and total value of an ecosystem. Valuation of fungi is essential, as it will contribute toward better decision making in policy appraisals considering the full costs and benefits to the natural environment and human wellbeing, while providing policy development with new insights (DEFRA 2006 ). Monetary valuation, or monetarization, is used in translating measures of social and biophysical impacts into monetary units so that they can be compared against each other and against the costs and benefits already expressed in monetary units (Marquina et al. 2022 ). This is very helpful in assessing the global value of fungi. Humans tend to give value to expensive things because they enjoy the pleasure of price. Studies show that people tend to value expensive items over their cheaper or free counterparts (Schmidt et al. 2017 ). The global value of fungi in ecosystems is missing or is not recognized since we get them for free. No studies have been conducted yet to give the overall value of fungi. Under-representation of the services rendered by fungi can lead to an underestimation of the global significance of the contribution of fungi in forest ecosystems. Moreover, many potential applications of fungi in biotechnology and industry have not been fully explored. Fungi have the potential to generate money, especially in their role as a bioremediator, mycoinsecticide, mycofertilizer, and biofuel. Quantifying ecosystem services provided by fungi and giving them a monetary price can help appraise their value to be taken into account in policy making and development. Formal and comprehensive ecosystem assessment would require considerable investment but could substantially improve coordination between management bodies, such as legislators and beneficiaries that could help in the conservation of fungal resources both on the regional and global scale.
In this study, using data from a number of industries, we estimated the global market value of fungi at USD 54.57 trillion . We acknowledge this is the first attempt to evaluate fungi in monetary terms, and that future assessments building on our work will perhaps be able to improve on the accuracy of this value. We have had to assume the value of fungi for some key industries, especially the food and beverages industries, as the total value of the products produced in those industries was based on the assumption that the final products would not be able to be produced, without the contribution of fungi.
This study emphasizes that fungi have an enormous market value, having an undeniable impact on the global economy. Monetary valuation of global products and services by fungi as well as their roles in ecosystem functioning is crucial to drive policy regarding the conservation and industrialization of this natural resource. With the rapid advancement of new technologies and related industries, the market presence of fungi will only increase, and thus fungi are prone to unsuitable exploitation if not properly monitored and managed in the future. The huge financial value of fungi adds weight to the argument that landscapes need to be conserved in order to conserve the natural resources found within. We have only discovered a small percentage of the fungi found in nature; thus, it is highly probable that there are untold billions of dollars’ worth of fungal resources yet to be discovered, or lost if their habitats are destroyed.
Data availability
No data were generated for this study.
Abel EL (2001) The gin epidemic: much ado about what? Alcohol Alcohol 36:401–405. https://doi.org/10.1093/alcalc/36.5.401
Article CAS PubMed Google Scholar
Ache NT, Manju EB, Lawrence MN, Tonjock RK (2021) Nutrient and mineral components of wild edible mushrooms from the Kilum-Ijim forest, Cameroon. Afr J Food Sci 15:152–161. https://doi.org/10.5897/ajfs2021.2089
Article CAS Google Scholar
Agbowuro AA, Huston WM, Gamble AB, Tyndall JDA (2018) Proteases and protease inhibitors in infectious diseases. Med Res Rev 38:1295–1331. https://doi.org/10.1002/med.21475
Agboyibor C, Kong W-B, Chen D et al (2018) Monascus pigments production, composition, bioactivity and its application: a review. Biocatal Agric Biotechnol 16:433–447. https://doi.org/10.1016/j.bcab.2018.09.012
Article Google Scholar
Agrawal N, Verma P, Shahi SK (2018) Degradation of polycyclic aromatic hydrocarbons (phenanthrene and pyrene) by the ligninolytic fungi Ganoderma lucidum isolated from the hardwood stump. Bioresour Bioprocess 5:11. https://doi.org/10.1186/s40643-018-0197-5
Ahnan-Winarno AD, Cordeiro L, Winarno FG et al (2021) Tempeh: a semicentennial review on its health benefits, fermentation, safety, processing, sustainability, and affordability. Compr Rev Food Sci Food Saf 20:1717–1767. https://doi.org/10.1111/1541-4337.12710
Akinfemiwa O, Zubair M, Muniraj T (2022) Amylase. StatPearls Publishing, Treasure Island
Google Scholar
Al Farraj DA, Hadibarata T, Elshikh MS et al (2020) Biotransformation and degradation pathway of pyrene by filamentous soil fungus Trichoderma sp. F03. Water Air Soil Pollut 231:168. https://doi.org/10.1007/s11270-020-04514-0
Alcohol Rehab Guide (2022) Types of alcohol. https://www.alcoholrehabguide.org/alcohol/types/#:~:text=Vodka%2C a liquor usually made,ABV in the United States. accessed 5 Sep 2022
Allied Market Research (2022a) Reishi mushroom market. https://www.alliedmarketresearch.com/reishi-mushroom-market-A10352 . accessed 23 Aug 2022
Allied Market Research (2022b) Beer market. https://www.alliedmarketresearch.com/beer-market . accessed 2 Sep 2022
Allied Market Research (2022c) Gin market. https://www.alliedmarketresearch.com/gin-market-A11469#:~:text=The global gin market was, forecast period 2021 to 2028. accessed 5 Sep 2022
Allied Market Research (2022d) Whiskey market. https://www.alliedmarketresearch.com/whiskey-market-A06652#:~:text=The global whiskey market was,share in the whiskey market. accessed 5 Sep 2022
Allied Market Research (2022e) Fungal protein market by type. https://www.alliedmarketresearch.com/fungal-protein-market-A12366 . accessed 15 Dec 2022c
Allwood JG, Wakeling LT, Bean DC (2021) Fermentation and the microbial community of Japanese koji and miso: a review. J Food Sci 86:2194–2207. https://doi.org/10.1111/1750-3841.15773
Ameen SM, Caruso G (2017) Lactic acid in the food industry. Springer, Cham
Book Google Scholar
Aneesh PA, Ajeeshkumar KK, Lekshmi RGK et al (2022) Bioactivities of astaxanthin from natural sources, augmenting its biomedical potential: A review. Trends Food Sci Technol 125:81–90. https://doi.org/10.1016/j.tifs.2022.05.004
Anke T, Werle A, Bross M, Steglich W (1977) The strobilurins-new antifungal antibiotics from the basidiomycete Strobilurus tenacellus (Pers. Ex Fr.) Sing. J Antibiotics 30:806–810. https://doi.org/10.7164/antibiotics.30.806
Antunes F, Marçal S, Taofiq O et al (2020) Valorization of mushroom by-products as a source of value-added compounds and potential applications. Molecules 25:2672. https://doi.org/10.3390/molecules25112672
Article CAS PubMed PubMed Central Google Scholar
Asbel LE, Levison ME (2000) Cephalosporins, carbapenems, and monobactams. Infect Dis Clin North Am 14:435–447. https://doi.org/10.1016/S0891-5520(05)70256-7
Attias N, Danai O, Abitbol T et al (2020) Mycelium bio-composites in industrial design and architecture: comparative review and experimental analysis. J Clean Prod 246:119037. https://doi.org/10.1016/j.jclepro.2019.119037
Avalos J, Carmen Limón M (2015) Biological roles of fungal carotenoids. Curr Genet 61:309–324. https://doi.org/10.1007/s00294-014-0454-x
Badalyan SM, Rapior S (2021) The neurotrophic and neuroprotective potential of macrofungi. In: Agrawal DC, Dhanasekaran M (eds) Medicinal herbs and fungi. Springer, Singapore, pp 37–77
Chapter Google Scholar
Badalyan S, Badalyan S, Barkhudaryan A (2021) The cardioprotective properties of Agaricomycetes mushrooms growing in the territory of Armenia (review). Int J Med Mushrooms 23:21–31. https://doi.org/10.1615/IntJMedMushrooms.2021038280
Article PubMed Google Scholar
Badalyan SM, Barkhudaryan A, Rapior S (2022) Medicinal macrofungi as cosmeceuticals: a review. Int J Med Mushrooms 24:1–13. https://doi.org/10.1615/IntJMedMushrooms.2022043124
Bandara AR, Rapior S, Bhat DJ et al (2015) Polyporus umbellatus , an edible-medicinal cultivated mushroom with multiple developed health-care products as food, medicine and cosmetics: a review. Cryptogam Mycol 36:3–42. https://doi.org/10.7872/crym.v36.iss1.2015.3
Bandara AR, Karunarathna SC, Mortimer PE et al (2017) First successful domestication and determination of nutritional and antioxidant properties of the red ear mushroom Auricularia thailandica (Auriculariales, Basidiomycota). Mycol Prog 16:1029–1039. https://doi.org/10.1007/s11557-017-1344-7
Barros L, Cruz T, Baptista P et al (2008) Wild and commercial mushrooms as source of nutrients and nutraceuticals. Food Chem Toxicol 46:2742–2747. https://doi.org/10.1016/j.fct.2008.04.030
Batt CA, Lou TM (2014) Encyclopedia of food microbiology, 2nd edn. Academic Press, Massachusetts
BBC (2022) Brandy. https://www.bbc.co.uk/food/brandy . accessed 5 Sep 2022
Bentley R (2000) Mycophenolic acid: A one hundred year odyssey from antibiotic to immunosuppressant. Chem Rev 100:3801–3826. https://doi.org/10.1021/cr990097b
Benz F, Knüsel F, Nüesch J et al (1974) Stoffwechselprodukte von Mikroorganismen 143. Mitteilung. Echinocandin B, ein neuartiges Polypeptid-Antibioticum aus Aspergillus nidulans var. echinulatus : Isolierung und Bausteine. Helv Chim Acta 57:2459–2477. https://doi.org/10.1002/hlca.19740570818
Berruti A, Lumini E, Balestrini R, Bianciotto V (2016) Arbuscular mycorrhizal fungi as natural biofertilizers: let’s benefit from past successes. Front Microbiol 6:1559. https://doi.org/10.3389/fmicb.2015.01559
Article PubMed PubMed Central Google Scholar
Bhalla TC, Savitri (2017) Yeasts and traditional fermented foods and beverages. In: Satyanarayana T, Kunze G (eds) Yeast diversity in human welfare. Springer, Singapore, pp 53–82
Bills GF, Gloer JB (2016) Biologically active secondary metabolites from the fungi. Microbiol Spectr 4:1–32. https://doi.org/10.1128/microbiolspec.FUNK-0009-2016
Bitting S, Derme T, Lee J et al (2022) Challenges and opportunities in scaling up architectural applications of mycelium-based materials with digital fabrication. Biomimetics 7:44. https://doi.org/10.3390/biomimetics7020044
Blanco P (1999) Production of pectic enzymes in yeasts. FEMS Microbiol Lett 175:1–9. https://doi.org/10.1016/S0378-1097(99)00090-7
Bockstael N (2007) Environmental and resource valuation with revealed preferences. Springer, Dordrecht
Brick J (2022) The world’s best rum comes from these countries. https://www.thrillist.com/drink/nation/best-rum-regions-worlds-best-rum . accessed 5 Sep 2022
Britannica (2022a) Types of beer. https://www.britannica.com/topic/beer/Types-of-beer . accessed 31 Aug 2022
Britannica (2022b) Learn how to make vodka. https://www.britannica.com/video/179764/Overview-vodka#:~:text=Traditionally%2C vodka is made from,the distillation process can begin. accessed 1 Sep 2022
Brown AG, Butterworth D, Cole M et al (1976) Naturally-occurring beta-lactamase inhibitors with antibacterial activity. J Antibiotics 29:668–669. https://doi.org/10.7164/antibiotics.29.668
Bulkan G, Ferreira JA, Taherzadeh MJ (2020) Removal of organic micro-pollutants using filamentous fungi. In: Varjani S, Pandey A, Tyagi RD, Nho HH, Larroche C (eds) Current developments in biotechnology and bioengineering. Elsevier, Amsterdam, pp 363–395
Business Research Insights (2022) Laccase market size, share, growth, and industry analysis by type. https://www.businessresearchinsights.com/market-reports/laccase-market-101221 . accessed 14 Feb 2023
Cairns TC, Nai C, Meyer V (2018) How a fungus shapes biotechnology: 100 years of Aspergillus niger research. Fungal Biol Biotechnol 5:13. https://doi.org/10.1186/s40694-018-0054-5
Caro Y, Venkatachalam M, Lebeau J et al (2017) Pigments and colorants from filamentous fungi. In: Mérillon JM, Ramawat KG (eds) Fungal metabolites. Springer, Cham, pp 499–568
Carocho M, Morales P, Ferreira ICFR (2018) Antioxidants: reviewing the chemistry, food applications, legislation and role as preservatives. Trends Food Sci Technol 71:107–120. https://doi.org/10.1016/j.tifs.2017.11.008
Carvalho  (2016) Yeasts and chocolate, the new “BFFs.” https://yeastcell.eu/2016/02/16/yeasts-and-chocolate-the-new-bffs/ . accessed 5 Sep 2022
Cascone R (2008) Biobutanol-a replacement for bioethanol? Chem Eng Prog 104:S4–S9
CAS Google Scholar
Castillo Martinez FA, Balciunas EM, Salgado JM et al (2013) Lactic acid properties, applications and production: a review. Trends Food Sci Technol 30:70–83. https://doi.org/10.1016/j.tifs.2012.11.007
Cerimi K, Akkaya KC, Pohl C et al (2019) Fungi as source for new bio-based materials: a patent review. Fungal Biol Biotechnol 6:17. https://doi.org/10.1186/s40694-019-0080-y
Chadni Z, Rahaman MH, Jerin I et al (2017) Extraction and optimisation of red pigment production as secondary metabolites from Talaromyces verruculosus and its potential use in textile industries. Mycology 8:48–57. https://doi.org/10.1080/21501203.2017.1302013
Chang ST, Miles P (2004a) Pleurotus : a mushroom of broad adaptability. Mushrooms: cultivation, nutritional value, medicinal effect, and environmental impact, 2nd edn. CRC Press, Boca Raton, pp 315–325
Chang ST, Miles PG (2004b) Mushrooms: cultivation, nutritional value, medicinal effect, and environmental impact, 2nd edn. CRC Press, Boca Raton
ChEBI (2022) Lovastatin. http://www.ebi.ac.uk/chebi/searchId.do?chebiId=CHEBI:40303 . accessed 20 Aug 2022
Chelala BL, Chacha M, Matemu A (2014) Wild edible mushroom value chain for improved livelihoods in Southern Highlands of Tanzania. Am J Res Commun 2:1–14
Cheng T, Kolařík M, Quijada L, Stadler M (2022) A re-assessment of Taxomyces andreanae , the alleged taxol-producing fungus, using comparative genomics. IMA Fungus 13:17. https://doi.org/10.1186/s43008-022-00103-4
Chourasia R, Abedin MM, Chiring Phukon L et al (2021) Biotechnological approaches for the production of designer cheese with improved functionality. Compr Rev Food Sci Food Saf 20:960–979. https://doi.org/10.1111/1541-4337.12680
Chowdhury M, Hussain MD, Chung SO et al (2016) Agarwood manufacturing: a multidisciplinary opportunity for economy of Bangladesh-a review. Agric Eng Int CIGR J 18:171–178
Christiana NO (2016) Production of food colourants by filamentous fungi. African J Microbiol Res 10:960–971. https://doi.org/10.5897/AJMR2016.7904
Chugh M, Kumar L, Bharadvaja N (2022) Chapter 4-Fungal diversity in the bioremediation of toxic effluents. In: Rodriguez-Couto S, Shah M (eds) Development in wastewater treatment research and processes. Elsevier, Amsterdam, pp 61–88
Clemmensen KE, Bahr A, Ovaskainen O et al (2013) Roots and associated fungi drive long-term carbon sequestration in boreal forest. Science 339:1615–1618. https://doi.org/10.1126/science.1231923
Cohen N, Cohen J, Asatiani MD et al (2014) Chemical composition and nutritional and medicinal value of fruit bodies and submerged cultured mycelia of culinary-medicinal higher basidiomycetes mushrooms. Int J Med Mushrooms 16:273–291. https://doi.org/10.1615/IntJMedMushr.v16.i3.80
Colorado State University Extension (2023) Functional Foods for Health–9.391. https://extension.colostate.edu/topic-areas/nutrition-food-safety-health/functional-foods-for-health-9-391/#:~:text=The term functional foods is,chronic disease and condition development. accessed 8 May 2023
Conway J (2022) Vodka industry- statistics & facts. In: Statista. https://www.statista.com/topics/3741/vodka-industry/#:~:text=World market,9 liter cases in 2020. accessed 5 Sep 2022
Copetti MV (2019) Fungi as industrial producers of food ingredients. Curr Opin Food Sci 25:52–56. https://doi.org/10.1016/j.cofs.2019.02.006
Corrêa RCG, da Silva BP, Castoldi R et al (2016) Spent mushroom substrate of Pleurotus pulmonarius : a source of easily hydrolyzable lignocellulose. Folia Microbiol (praha) 61:439–448. https://doi.org/10.1007/s12223-016-0457-8
Cutler WD II, Bradshaw AJ, Dentinger BTM (2021) What’s for dinner this time?: DNA authentication of “wild mushrooms” in food products sold in the USA. PeerJ 9:e11747. https://doi.org/10.7717/peerj.11747
Data Bridge Market Research (2022) Global echinocandins market – Industry trends and forecast to 2029. https://www.databridgemarketresearch.com/reports/global-echinocandins-market . accessed 3 Feb 2023
Dataintelo (2022a) Global fungal protease market by type. https://dataintelo.com/report/global-fungal-protease-market/ . accessed 31 Oct 2022
Dataintelo (2022b) Global galactosidase market by type. https://dataintelo.com/report/global-galactosidase-market/ . accessed 29 Oct 2022
de Almeida Silva Vilela J, de Figueiredo Vilela L, Ramos CL, Schwan RF (2020) Physiological and genetic characterization of indigenous Saccharomyces cerevisiae for potential use in productions of fermented maize-based-beverages. Braz J Microbiol 51:1297–1307. https://doi.org/10.1007/s42770-020-00271-8
De Filippis F, Troise AD, Vitaglione P, Ercolini D (2018) Different temperatures select distinctive acetic acid bacteria species and promotes organic acids production during Kombucha tea fermentation. Food Microbiol 73:11–16. https://doi.org/10.1016/j.fm.2018.01.008
De Silva DD, Rapior S, Sudarman E et al (2013) Bioactive metabolites from macrofungi: ethnopharmacology, biological activities and chemistry. Fungal Divers 62:1–40. https://doi.org/10.1007/s13225-013-0265-2
Debnath S, Debnath B, Das P, Saha AK (2019) Review on an ethnomedicinal practices of wild mushrooms by the local tribes of India. J Appl Pharm Sci 9:144–156. https://doi.org/10.7324/JAPS.2019.90818
DEFRA (2006) An introductory guide to valuing ecosystem services. London
Demain AL, Sanchez S (2009) Microbial drug discovery: 80 years of progress. J Antibiotics 62:5–16. https://doi.org/10.1038/ja.2008.16
Derbyshire E (2022) Fungal-derived mycoprotein and health across the lifespan: a narrative review. J Fungi 8:653. https://doi.org/10.3390/jof8070653
Derbyshire EJ, Delange J (2021) Fungal protein – What is it and what is the health evidence? A systematic review focusing on mycoprotein. Front Sustain Food Syst 5:581682. https://doi.org/10.3389/fsufs.2021.581682
Diallo I, Morel S, Vitou M, et al (2020) Ergosterol and amino acids contents of culinary-medicinal Shiitake from various culture conditions. Proceedings 70:78. https://doi.org/10.3390/foods_2020-07702
Dinesh Babu P, Bhakyaraj R, Vidhyalakshmi R (2009) A low cost nutritious food “Tempeh”-a review. World J Dairy Food Sci 4:22–27
Domínguez-Núñez JA, Berrocal-Lobo M, Albanesi A (2020) Ectomycorrhizal fungi as biofertilizers in forestry. In: Mirmajlessi SM, Radhakrishnan R (eds) Biostimulants in plant science. IntechOpen, Rijeka
Dong C, Guo S, Wang W, Liu X (2015) Cordyceps industry in China. Mycology 6:121–129. https://doi.org/10.1080/21501203.2015.1043967
Dörsam S, Fesseler J, Gorte O et al (2017) Sustainable carbon sources for microbial organic acid production with filamentous fungi. Biotechnol Biofuels 10:242. https://doi.org/10.1186/s13068-017-0930-x
Dowdells C, Jones RL, Mattey M et al (2010) Gluconic acid production by Aspergillus terreus . Lett Appl Microbiol 51:252–257. https://doi.org/10.1111/j.1472-765X.2010.02890.x
Drugbank Online (2022a) Griseofulvin. https://go.drugbank.com/drugs/DB00400 . accessed 21 Aug 2022
Drugbank Online (2022b) Fingolimod. https://go.drugbank.com/drugs/DB08868 . Accessed 22 Aug 2022
Dufossé L (2019) Pigments, microbial. In: Schmidt T (ed) Encyclopedia of microbiology, 4th edn. Academic Press, Oxford, pp 579–594
Dufossé L, Fouillaud M, Caro Y et al (2014) Filamentous fungi are large-scale producers of pigments and colorants for the food industry. Curr Opin Biotechnol 26:56–61. https://doi.org/10.1016/j.copbio.2013.09.007
Ecovative Design LLC (2023) Ecovative design. https://www.ecovative.com/ . accessed 2 Feb 2023
Edy N, Barus HN, Finkeldey R, Polle A (2022) Host plant richness and environment in tropical forest transformation systems shape arbuscular mycorrhizal fungal richness. Front Plant Sci 13:1004097. https://doi.org/10.3389/fpls.2022.1004097
El-Gendi H, Saleh AK, Badierah R et al (2021) A comprehensive insight into fungal enzymes: structure, classification, and their role in mankind’s challenges. J Fungi 8:23. https://doi.org/10.3390/jof8010023
El Enshasy HA, Hatti-Kaul R (2013) Mushroom immunomodulators: unique molecules with unlimited applications. Trends Biotechnol 31:668–677. https://doi.org/10.1016/j.tibtech.2013.09.003
Embrandiri A, Kiyasudeen SK, Rupani PF, Ibrahim MH (2016) Environmental xenobiotics and its effects on natural ecosystem. In: Singh A, Prasad S, Singh R (eds) Plant responses to xenobiotics. Springer, Singapore, pp 1–18
Endo A (1979) Monacolin K, a new hypocholesterolemic agent produced by a Monascus species. J Antibiotics 32:852–854. https://doi.org/10.7164/antibiotics.32.852
Endo A (2008) A gift from nature: the birth of the statins. Nat Med 14:1050–1052. https://doi.org/10.1038/nm1008-1050
Endo A (2010) A historical perspective on the discovery of statins. Proc Jpn Acad Ser B Phys Biol Sci 86(5):484−463. https://doi.org/10.2183/pjab.86.484
Endo A, Kuroda M, Tsujita Y (1976) ML-236A, ML-236B, and ML-236C, new inhibitors of cholesterogenesis produced by Penicillium citrinium . J Antibiotics 29:1346–1348. https://doi.org/10.7164/antibiotics.29.1346
Espinel-Ingroff A, Dannaoui E (2021) Special issue: antifungal agents recently approved or under development. J Fungi 7:239. https://doi.org/10.3390/jof7030239
European Medicines Agency (2013) Fourth European surveillance of veterinary antimicrobial consumption (ESVAC) report. Sales of veterinary antimicrobial agents in 26 EU/EEA countries in 2012. Eur Med Agency, Amsterdam
FAO (2015) FAO coffee pocketbook. http://www.fao.org/3/a-i4985e.pdf . accessed 5 Sep 2022
FAOSTAT (2022a) Mushroom and truffles. https://www.fao.org/faostat/en/#search/Mushrooms and truffles. accessed 13 May 2022
FAOSTAT (2022b) Mushroom & truffles: Producer prices. https://www.fao.org/faostat/en/#search/mushroom . accessed 10 Jan 2023
Feng Y, Shao Y, Chen F (2012) Monascus pigments. Appl Microbiol Biotechnol 96:1421–1440. https://doi.org/10.1007/s00253-012-4504-3
Fernandes P (2016) Fusidic acid: A bacterial elongation factor inhibitor for the oral treatment of acute and chronic staphylococcal infections. Cold Spring Harb Perspect Med 6:a025437. https://doi.org/10.1101/cshperspect.a025437
Fior Market (2022) Alpha-amylase baking enzyme market by source. https://www.fiormarkets.com/report/alpha-amylase-baking-enzyme-market-by-source-fungi-bacteria-396099.html . accessed 29 Oct 2022
Flores-Gallegos AC, Delgado-García M, Ascacio-Valdés JA et al (2019) Hydrolases of halophilic origin with importance for the food industry. In: Kuddos M (ed) Enzymes in food biotechnology. Elsevier, Amsterdam, pp 197–219
Food Data Central (2019) Mushroom raw. https://fdc.nal.usda.gov/fdc-app.html#/food-details/169251/nutrients
Fortune Business Insight (2022a) Fermented food and drink market. https://www.fortunebusinessinsights.com/fermented-food-and-drinks-market-103255 . accessed 5 Sep 2022
Fortune Business Insight (2022b) Wine market. https://www.fortunebusinessinsights.com/wine-market-102836 . accessed 4 Sep 2022
Fortune Business Insight (2022c) Carotenoids market size, share & Covid-impact analysis, by type. https://www.fortunebusinessinsights.com/industry-reports/carotenoids-market-100180 . accessed 2 Nov 2022
Free SJ (2013) Fungal cell wall organization and biosynthesis. Adv Genet 81:33–82. https://doi.org/10.1016/B978-0-12-407677-8.00002-6
Frutos P, Martínez Peña F, Ortega Martínez P, Esteban S (2009) Estimating the social benefits of recreational harvesting of edible wild mushrooms using travel cost methods. For Syst 18:235. https://doi.org/10.5424/fs/2009183-01065
Fu TT, Shen L (2022) Ergothioneine as a natural antioxidant against oxidative stress-related diseases. Front Pharmacol 13:850813. https://doi.org/10.3389/fphar.2022.850813
Future Market Insights (2022a) Citric acid market. https://www.futuremarketinsights.com/reports/citric-acid-market . accessed 18 Sep 2022
Future Market Insights (2022b) Miso market. https://www.technavio.com/report/miso-market-industry-analysis . accessed 31 Oct 2022
Future Market Insights (2022c) Invertase market outlook (2022c-2032). https://www.futuremarketinsights.com/reports/invertase-market . accessed 29 Oct 2022
Future Market Insights (2022d) Cellulases market. https://www.futuremarketinsights.com/reports/cellulase-market . accessed 14 Feb 2023
Future Market Insights (2022e) Lipase market. https://www.futuremarketinsights.com/reports/lipase-market . accessed 14 Feb 2023
Galappaththi MCA, Patabendige NM, Premarathne BM et al (2022) A review of Ganoderma triterpenoids and their bioactivities. Biomolecules 13:24. https://doi.org/10.3390/biom13010024
Gandjar I (1999) Fermented foods | Fermentations of the far East. In: Robinson R (ed) Encyclopedia of food microbiology. Elsevier, Oxford, pp 767–773
Gao YG, Selmer M, Dunham CM et al (2009) The structure of the ribosome with elongation factor G trapped in the posttranslocational state. Science 326:694–699. https://doi.org/10.1126/science.1179709
Gentili F, Jumpponen A (2006) Potential and possible uses of bacterial and fungal biofertilizers. In: Rai M (ed) Handbook of microbial biofertilizers. CRC Press, Florida, pp 29–56
Ghannoum M, Arendrup MC, Chaturvedi VP et al (2020) Ibrexafungerp: A novel oral triterpenoid antifungal in development for the treatment of Candida auris infections. Antibiotics 9:539. https://doi.org/10.3390/antibiotics9090539
Gibson B, Geertman J-MA, Hittinger CT et al (2017) New yeasts—new brews: modern approaches to brewing yeast design and development. FEMS Yeast Res 17:fox038. https://doi.org/10.1093/femsyr/fox038
Global Market Insights (2022a) Onychomycosis treatment market size. https://www.gminsights.com/industry-analysis/onychomycosis-treatment-market . accessed 22 Aug 2022
Global Market Insights (2022b) Gluconic acid market. https://www.gminsights.com/industry-analysis/gluconic-acid-market . accessed 21 Sep 2022
Global Market Insights (2022c) Global beta glucan market. https://www.gminsights.com/industry-analysis/beta-glucans-market . accessed 13 Nov 2022
Globe Newswire (2022a) Brandy market growth segments 2022a. https://www.globenewswire.com/en/news-release/2022/01/31/2375510/0/en/Brandy-Market-Growth-Segments-2022-Trends-Regional-Analysis-Top-Players-Revenue-USD-25320-million-Forecast-by-2027-Report-by-Absolute-Reports.html#:~:text= The global Brandy market was,1. accessed 4 Sep 2022
Globe Newswire (2022b) Fungal protein market worth $397.5 million by 2029. https://www.globenewswire.com/en/news-release/2022b/06/22/2466987/0/en/Fungal-Protein-Market-Worth-397-5-Million-by-2029-Exclusive-Report-by-Meticulous-Research.html . accessed 11 Nov 2022
GlobeNewswire (2022) SCYNEXIS reports third quarter 2022 financial results and provides corporate update. https://www.globenewswire.com/en/news-release/2022/11/09/2551800/0/en/SCYNEXIS-Reports-Third-Quarter-2022-Financial-Results-and-Provides-Corporate-Update.html . accessed 3 Feb 2023
Godtfredsen WO, Jahnsen S, Lorck H et al (1962) Fusidic acid: a new antibiotic. Nature 193:987–987. https://doi.org/10.1038/193987a0
Goldberg I, Rokem JS (2009) Organic and fatty acid production, microbial. In: Schaechter M (ed) Encyclopedia of microbiology, 3rd edn. Academic Press, Cambridge, pp 421–442
Gopalakrishnan C, Pawar RS, Bhutani KK (2005) Development of Agaricus bisporus as a nutraceutical of tomorrow. Acta Hortic 680:45–47. https://doi.org/10.17660/ActaHortic.2005.680.5
Gosio B (1893) Sperimentate su culture pure di bacilli del carbonchio demonstrarano notevole potere antisettica. CR Acad Med Torino 61:484
Gosio B (1896) Richerche batteriologiche e chemiche sulle alterazoni del mais. Riv D’igiene Sanita Pub Ann 7:825–849
Graham C (2022e) What is tequila? https://www.thespruceeats.com/all-about-tequila-760706 . accessed 5 Sep 2022
Grand View Research (2022a) Chocolate market size, share & trends analysis report by product. https://www.grandviewresearch.com/industry-analysis/chocolate-market#:~:text=Report Overview,4.6%25 from 2020 to 2027. accessed 4 Sep 2022
Grand View Research (2022b) Lactase market. https://www.grandviewresearch.com/industry-analysis/lactase-market . accessed 29 Oct 2022
Grand View Research (2023a) GVR report cover astaxanthin market size, share & trends report astaxanthin market size, share & trends analysis report by product. https://www.grandviewresearch.com/industry-analysis/global-astaxanthin-market . accessed 14 Jan 2023
Grand View Research (2023b) Yeast beta-glucan market size, share & trends analysis report by application. https://www.grandviewresearch.com/industry-analysis/yeast-beta-glucan-market-report . accessed 19 Jan 2023
Grand View Research (2023c) Bioremediation market size, share & trends analysis report by type. https://www.grandviewresearch.com/industry-analysis/bioremediation-market-report . accessed 12 Jan 2023
Grewal HS, Kalra KL (1995) Fungal production of citric acid. Biotechnol Adv 13:209–234. https://doi.org/10.1016/0734-9750(95)00002-8
GrownBio (2022) 100% natural alternative to plastic foam. https://www.grown.bio/ . accessed 2 Feb 2023
Grubb M, Vrolijk C, Brack D (1999) The Kyoto protocol: a guide and assessment. Royal Institute for International Affairs, London
Grujić M, Dojnov B, Potočnik I et al (2015) Spent mushroom compost as substrate for the production of industrially important hydrolytic enzymes by fungi Trichoderma spp. and Aspergillus niger in solid state fermentation. Int Biodeterior Biodegradation 104:290–298. https://doi.org/10.1016/j.ibiod.2015.04.029
Gupta AK, Foley KA, Versteeg SG (2017) New antifungal agents and new formulations against dermatophytes. Mycopathologia 182:127–141. https://doi.org/10.1007/s11046-016-0045-0
Gupta R, Gigras P, Mohapatra H et al (2003) Microbial α-amylases: a biotechnological perspective. Process Biochem 38:1599–1616. https://doi.org/10.1016/S0032-9592(03)00053-0
Gupta S, Summuna B, Gupta M, Annepu SK (2018) Edible mushrooms: cultivation, bioactive molecules, and health benefits. In: Mérillon JM, Ramawat K (eds) Bioactive molecules in food. Reference series in phytochemistry. Springer, Cham, pp 1–33
Gupta VK, Kubicek CP, Berrin J-G et al (2016) Fungal enzymes for bio-products from sustainable and waste biomass. Trends Biochem Sci 41:633–645. https://doi.org/10.1016/j.tibs.2016.04.006
Gurung N, Ray S, Bose S, Rai V (2013) A broader view: Microbial enzymes and their relevance in industries, medicine, and beyond. Biomed Res Int 2013:329121. https://doi.org/10.1155/2013/329121
Gutiérrez-Ríos HG, Suárez-Quiroz ML, Hernández-Estrada ZJ et al (2022) Yeasts as producers of flavor precursors during cocoa bean fermentation and their relevance as starter cultures: a review. Fermentation 8:331. https://doi.org/10.3390/fermentation8070331
Gygli G, van Berkel JH (2015) Oxizymes for biotechnology. Curr Biotechnol 4:100–110. https://doi.org/10.2174/2211550104666150423202036
Hadibarata T, Kristanti RA (2013) Biodegradation and metabolite transformation of pyrene by basidiomycetes fungal isolate Armillaria sp. F022. Bioprocess Biosyst Eng 36:461–468. https://doi.org/10.1007/s00449-012-0803-4
Hadibarata T, Teh ZC (2014) Optimization of pyrene degradation by white-rot fungus Pleurotus pulmonarius F043 and characterization of its metabolites. Bioprocess Biosyst Eng 37:1679–1684. https://doi.org/10.1007/s00449-014-1140-6
Haile S, Ayele A (2022) Pectinase from microorganisms and its industrial applications. Sci World J 2022:1–15. https://doi.org/10.1155/2022/1881305
Hamwenye KKN, Ueitele ISE, Kadhila NP et al (2022) Towards medicinal tea from untapped Namibian Ganoderma : Phenolics and in vitro antioxidant activity of wild and cultivated mushrooms. S Afr J Sci 118:9357. https://doi.org/10.17159/sajs.2022/9357
Handoyo T, Morita N (2006) Structural and functional properties of fermented soybean (Tempeh) by using Rhizopus oligosporus . Int J Food Prop 9:347–355. https://doi.org/10.1080/10942910500224746
Harder A, Pham T, Jarling R, et al (2011) Method for producing optically active, cyclic depsipeptides comprising lactic acid and phenyl lactic and having 24 ring atoms, using fungus strains of Rosellinia type, and further species of Xylariaceae (Patent No. WO2010072323A1). European Patent Office13/133,611
He J (2018) Harvest and trade of caterpillar mushroom ( Ophiocordyceps sinensis ) and the implications for sustainable use in the Tibet Region of Southwest China. J Ethnopharmacol 221:86–90. https://doi.org/10.1016/j.jep.2018.04.022
He L, Mao Y, Zhang L et al (2017) Functional expression of a novel α-amylase from Antarctic psychrotolerant fungus for baking industry and its magnetic immobilization. BMC Biotechnol 17:22. https://doi.org/10.1186/s12896-017-0343-8
Helaly SE, Thongbai B, Stadler M (2018) Diversity of biologically active secondary metabolites from endophytic and saprotrophic fungi of the ascomycete order Xylariales. Nat Prod Rep 35:992–1014. https://doi.org/10.1039/C8NP00010G
Hernández VA, Galleguillos F, Thibaut R, Müller A (2019) Fungal dyes for textile applications: testing of industrial conditions for wool fabrics dyeing. J Text Inst 110:61–66. https://doi.org/10.1080/00405000.2018.1460037
Ho CW, Lazim AM, Fazry S et al (2017) Varieties, production, composition and health benefits of vinegars: a review. Food Chem 221:1621–1630. https://doi.org/10.1016/j.foodchem.2016.10.128
Hong S-B, Kim D-H, Samson RA (2015) Aspergillus associated with meju, a fermented soybean starting material for traditional soy sauce and soybean paste in Korea. Mycobiology 43:218–224. https://doi.org/10.5941/MYCO.2015.43.3.218
Hoondal G, Tiwari R, Tewari R et al (2002) Microbial alkaline pectinases and their industrial applications: a review. Appl Microbiol Biotechnol 59:409–418. https://doi.org/10.1007/s00253-002-1061-1
Houbraken J, Frisvad JC, Samson RA (2011) Fleming’s penicillin producing strain is not Penicillium chrysogenum but P. rubens . IMA Fungus 2:87–95. https://doi.org/10.5598/imafungus.2011.02.01.12
Hu X, Robin S, O’Connell S et al (2010) Engineering of a fungal beta-galactosidase to remove product inhibition by galactose. Appl Microbiol Biotechnol 87:1773–1782. https://doi.org/10.1007/s00253-010-2662-8
Hübner MP, Townson S, Gokool S et al (2021) Evaluation of the in vitro susceptibility of various filarial nematodes to emodepside. Int J Parasitol Drugs Drug Resist 17:27–35. https://doi.org/10.1016/j.ijpddr.2021.07.005
Hüttel W (2021) Echinocandins: structural diversity, biosynthesis, and development of antimycotics. Appl Microbiol Biotechnol 105:55–66. https://doi.org/10.1007/s00253-020-11022-y
Hyde KD, Bahkali AH, Moslem MA (2010) Fungi—an unusual source for cosmetics. Fungal Divers 43:1–9. https://doi.org/10.1007/s13225-010-0043-3
Hyde KD, Xu J, Rapior S et al (2019) The amazing potential of fungi: 50 ways we can exploit fungi industrially. Fungal Divers 97:1–136. https://doi.org/10.1007/s13225-019-00430-9
Hymery N, Vasseur V, Coton M et al (2014) Filamentous fungi and mycotoxins in cheese: a review. Compr Rev Food Sci Food Saf 13:437–456. https://doi.org/10.1111/1541-4337.12069
Imarc (2022a) Cephalosporin market: Global industry trends, share, size, growth, opportunity and forecast 2023–2028. https://www.imarcgroup.com/cephalosporin-market . accessed 3 Feb 2023
Imarc (2022b) Statin market: Global industry trends, share, size, growth, opportunity and forecast 2022b-2027. https://www.imarcgroup.com/statin-market . accessed 3 Feb 2023
Imarc (2022c) Global vinegar market outlook 2022c- 2027. https://www.imarcgroup.com/vinegar-manufacturing-plant#:~:text=The global vinegar market was,US%24 2.27 Billion in 2021. accessed 5 Sep 2022
Imarc (2023) Bakery products market: Global industry trends, share, size, growth, opportunity and forecast 2023–2028. https://www.imarcgroup.com/bakery-products-market#:~:text=The global bakery products market,3.7%25 during 2023–2028. accessed 25 Jan 2023
Infita (2022) Uses of invertase enzymes. https://infinitabiotech.com/blog/uses-of-invertase-enzyme/#:~:text=Invertase is one such enzyme,to extend their shelf life. accessed 29 Oct 2022
InsightAce Analytic (2021) Global mycelium market. https://www.insightaceanalytic.com/report/global-mycelium-market-/1219 . accessed 21 Jun 2022
International Trade Center (2022) Trade map. https://www.trademap.org/Index.aspx . accessed 5 Sep 2022
IPCC (2005) Chapter 9: Implications of carbon dioxide capture and storage for greenhouse gas inventories and accounting. In: Hiraishi T, Miguez JD (eds) IPCC special report on carbon dioxide capture and storage. pp 363–379
Iqbal Z, Han LC, Soares-Sello AM et al (2018) Investigations into the biosynthesis of the antifungal strobilurins. Org Biomol Chem 16:5524–5532. https://doi.org/10.1039/c8ob00608c
Ito K, Matsuyama A (2021) Koji molds for Japanese soy sauce brewing: characteristics and key enzymes. J Fungi 7:658. https://doi.org/10.3390/jof7080658
Iwamoto T, Fujie A, Nitta K et al (1994) WF11899A, B and C, novel antifungal lipopeptides. II. Biological properties. J Antibiotics 47:1092–1097. https://doi.org/10.7164/antibiotics.47.1092
Jacinto-Azevedo B, Valderrama N, Henríquez K et al (2021) Nutritional value and biological properties of Chilean wild and commercial edible mushrooms. Food Chem 356:129651. https://doi.org/10.1016/j.foodchem.2021.129651
Jain A, Utley L, Parr TR et al (2018) Tebipenem, the first oral carbapenem antibiotic. Expert Rev Anti Infect Ther 16:513–522. https://doi.org/10.1080/14787210.2018.1496821
Jallow S, Govender NP (2021) Ibrexafungerp: a first-in-class oral triterpenoid glucan synthase inhibitor. J Fungi 7:163. https://doi.org/10.3390/jof7030163
Jang K-Y, Cho S-M, Seok S-J et al (2009) Screening of biodegradable function of indigenous ligno-degrading mushroom using dyes. Mycobiology 37:53. https://doi.org/10.4489/MYCO.2009.37.1.053
Jayabalan R, Malbaša RV, Lončar ES et al (2014) A review on Kombucha tea—microbiology, composition, fermentation, beneficial effects, toxicity, and tea fungus. Compr Rev Food Sci Food Saf 13:538–550. https://doi.org/10.1111/1541-4337.12073
Jayani RS, Saxena S, Gupta R (2005) Microbial pectinolytic enzymes: a review. Process Biochem 40:2931–2944. https://doi.org/10.1016/j.procbio.2005.03.026
Jesus LFMC, Guimarães LHS (2021) Production of β-galactosidase by Trichoderma sp. through solid-state fermentation targeting the recovery of galactooligosaccharides from whey cheese. J Appl Microbiol 130:865–877. https://doi.org/10.1111/jam.14805
Jiang W, Hu J-W, He X-R et al (2021) Statins: a repurposed drug to fight cancer. J Exp Clin Cancer Res 40:241. https://doi.org/10.1186/s13046-021-02041-2
Johnston RJ, Wainger LA (2015) Benefit transfer for ecosystem service valuation: an introduction to theory and methods. In: Johnston RJ, Rolfe J, Rosenberger RS, Brouwer R (eds) Benefit transfer of environmental and resource values. Springer, Dordrecht, pp 237–273
Jones M, Mautner A, Luenco S et al (2020) Engineered mycelium composite construction materials from fungal biorefineries: a critical review. Mater Des 187:108397. https://doi.org/10.1016/j.matdes.2019.108397
Joseph S (2019) Nabriva prices two pneumonia antibiotic versions at over $200 per day. https://www.reuters.com/article/us-nabriva-fda-idUSKCN1V91QQ . accessed 13 Feb 2023
Kaewchai S, Soytong K, Hyde KD (2009) Mycofungicides and fungal biofertilizers. Fungal Divers 38:25–50
Kahan JS, Kahan FM, Goegelman R et al (1979) Thienamycin, a new beta-lactam antibiotic I. Discovery, taxonomy, isolation and physical properties. J Antibiotics 32:1–12. https://doi.org/10.7164/antibiotics.32.1
Kalač P (2016) Health-stimulating compounds and effects. In: Kalač P (ed) Edible mushrooms. Elsevier, Amsterdam, pp 137–153
Kalra R, Conlan XA, Goel M (2020) Fungi as a potential source of pigments: harnessing filamentous fungi. Front Chem 8:369. https://doi.org/10.3389/fchem.2020.00369
Kango N, Jana UK, Choukade R (2019) Fungal enzymes: sources and biotechnological applications. In: Satyanarayana T, Deshmukh SK, Deshpande MV (eds) Advancing frontiers in mycology & mycotechnology. Springer, Singapore, pp 515–538
Kanny D, Brewer RD, Mesnick JB et al (2015) Vital signs: alcohol poisoning deaths-United States, 2010–2012. MMWR Morb Mortal Wkly Rep 63:1238–1242
PubMed PubMed Central Google Scholar
Kant Raut J (2019) Current status, challenges and prospects of mushroom industry in Nepal. Int J Agric Econ 4:154. https://doi.org/10.11648/j.ijae.20190404.13
Karana E, Blauwhoff D, Hultink EJ, Camere S (2018) When the material grows: a case study on designing (with) mycelium-based materials. Int J Des 12:119–136
Karasova P, Spiwok V, Malá Š et al (2002) Beta-galactosidase activity in psychrotrophic microorganisms and their potential use in food industry. Czech J Food Sci 20:43. https://doi.org/10.17221/3508-CJFS
Karima B, Samia T (2020) Native arbuscular mycorrhizal fungi and agro-industries in arid lands: productions, applications strategies and challenges. In: Radhakrishnan R (ed) mycorrhizal fungi. IntechOpen, Rijeka
Kawasaki Y (2009) Mizoribine: a new approach in the treatment of renal disease. Clin Dev Immunol 2009:681482. https://doi.org/10.1155/2009/681482
Keller NP (2019) Fungal secondary metabolism: regulation, function and drug discovery. Nat Rev Microbiol 17:167–180. https://doi.org/10.1038/s41579-018-0121-1
Kluepfel D, Bagli J, Baker H et al (1972) Myriocin, a new antifungal antibiotic from Myriococcum albomyces . J Antibiotics 25:109–115. https://doi.org/10.7164/antibiotics.25.109
Koné MK, Guéhi ST, Durand N et al (2016) Contribution of predominant yeasts to the occurrence of aroma compounds during cocoa bean fermentation. Food Res Int 89:910–917. https://doi.org/10.1016/j.foodres.2016.04.010
Kotowski M (2016) Differences between European regulations on wild mushroom commerce and actual trends in wild mushroom picking. Slovak Ethnol 64:169–178
Koul B, Farooq B (2020) Chapter 12-Mycotechnology: utility of fungi in food and beverage industries. In: Singh J, Gehlot P (eds) New and future developments in microbial biotechnology and bioengineering. Elsevier, Amsterdam, pp 133–153
Krücken J, Holden-Dye L, Keiser J et al (2021) Development of emodepside as a possible adulticidal treatment for human onchocerciasis-The fruit of a successful industrial-academic collaboration. PLoS Pathog 17:e1009682. https://doi.org/10.1371/journal.ppat.1009682
Kulshreshtha S, Mathur N, Bhatnagar P (2014) Mushroom as a product and their role in mycoremediation. AMB Express 4:29. https://doi.org/10.1186/s13568-014-0029-8
Kulshrestha S, Tyagi P, Sindhi V, Yadavilli KS (2013) Invertase and its applications–a brief review. J Pharm Res 7:792–797. https://doi.org/10.1016/j.jopr.2013.07.014
Lagashetti AC, Dufossé L, Singh SK, Singh PN (2019) Fungal pigments and their prospects in different industries. Microorganisms 7:604. https://doi.org/10.3390/microorganisms7120604
Lahue C, Madden AA, Dunn RR, Smukowski Heil C (2020) History and domestication of Saccharomyces cerevisiae in bread baking. Front Genet 11:584718. https://doi.org/10.3389/fgene.2020.584718
Lai T, Gao Y, Zhou S (2004) Global marketing of medicinal Ling Zhi mushroom Ganoderma lucidum (W.Curt.:Fr.) Lloyd (Aphyllophoromycetideae) products and safety concerns. Int J Med Mushrooms 6:189–194. https://doi.org/10.1615/IntJMedMushr.v6.i2.100
Laich F, Fierro F, Martín JF (2002) Production of penicillin by fungi growing on food products: identification of a complete penicillin gene cluster in Penicillium griseofulvum and a truncated cluster in Penicillium verrucosum . Appl Environ Microbiol 68:1211–1219. https://doi.org/10.1128/AEM.68.3.1211-1219.2002
Lateef A, Oloke J, Gueguim-Kana E, Raimi O (2012) Production of fructosyltransferase by a local isolate of Aspergillus niger in both submerged and solid substrate media. Acta Aliment 41:100–117. https://doi.org/10.1556/AAlim.41.2012.1.12
Lee WY, Park E-J, Ahn JK, Ka K-H (2009) Ergothioneine contents in fruiting bodies and their enhancement in mycelial cultures by the addition of methionine. Mycobiology 37:43. https://doi.org/10.4489/MYCO.2009.37.1.043
Lei W, Zhang G, Peng Q, Liu X (2015) Development of Ophiocordyceps sinensis through plant-mediated interkingdom host colonization. Int J Mol Sci 16:17482–17493. https://doi.org/10.3390/ijms160817482
Lenihan C, Tan JC (2020) Clinical management of the adult kidney transplant recipient. In: Yu ASL, Chertow GM, Luyckx VA et al (eds) Brenner and rector’s the kidney, 11th edn. Elsevier, Philadelphia, pp 2244–2287
Lessard M-H, Viel C, Boyle B et al (2014) Metatranscriptome analysis of fungal strains Penicillium camemberti and Geotrichum candidum reveal cheese matrix breakdown and potential development of sensory properties of ripened Camembert-type cheese. BMC Genomics 15:235. https://doi.org/10.1186/1471-2164-15-235
Li C, Zhu Y, Wang Y et al (1998) Monascus purpureus -fermented rice (red yeast rice): a natural food product that lowers blood cholesterol in animal models of hypercholesterolemia. Nutr Res 18:71–81. https://doi.org/10.1016/S0271-5317(97)00201-7
Li X, Lin X, Zhang J et al (2010) Degradation of polycyclic aromatic hydrocarbons by crude extracts from spent mushroom substrate and its possible mechanisms. Curr Microbiol 60:336–342. https://doi.org/10.1007/s00284-009-9546-0
Li H, Tian Y, Menolli N et al (2021) Reviewing the world’s edible mushroom species: a new evidence-based classification system. Compr Rev Food Sci Food Saf 20:1982–2014. https://doi.org/10.1111/1541-4337.12708
Liaud N, Giniés C, Navarro D et al (2014) Exploring fungal biodiversity: organic acid production by 66 strains of filamentous fungi. Fungal Biol Biotechnol 1:1. https://doi.org/10.1186/s40694-014-0001-z
Article PubMed Central Google Scholar
Lin X, Kück U (2022) Cephalosporins as key lead generation beta-lactam antibiotics. Appl Microbiol Biotechnol 106:8007–8020. https://doi.org/10.1007/s00253-022-12272-8
Lin AX, Chan G, Hu Y et al (2018) Internationalization of traditional Chinese medicine: current international market, internationalization challenges and prospective suggestions. Chin Med 13:9. https://doi.org/10.1186/s13020-018-0167-z
Lipsky JJ (1996) Mycophenolate mofetil. Lancet (london, England) 348:1357–1359. https://doi.org/10.1016/S0140-6736(96)10310-X
Liu Y, Li X, Kou Y (2020a) Ectomycorrhizal fungi: participation in nutrient turnover and community assembly pattern in forest ecosystems. Forests 11:453. https://doi.org/10.3390/f11040453
Liu Y, Luo M, Liu F et al (2020b) Effects of freeze drying and hot-air drying on the physicochemical properties and bioactivities of polysaccharides from Lentinula edodes . Int J Biol Macromol 145:476–483. https://doi.org/10.1016/j.ijbiomac.2019.12.222
Lobanovska M, Pilla G (2017) Penicillin’s discovery and antibiotic resistance: lessons for the future? Yale J Biol Med 90:135–145
CAS PubMed PubMed Central Google Scholar
Lu H, Lou H, Hu J et al (2020) Macrofungi: a review of cultivation strategies, bioactivity, and application of mushrooms. Compr Rev Food Sci Food Saf 19:2333–2356. https://doi.org/10.1111/1541-4337.12602
Magnuson JK, Lasure LL (2004) Organic acid production by filamentous fungi. In: Lange L (ed) Advances in fungal biotechnology for industry, agriculture, and medicine. Springer, Boston, pp 307–340
Maheshwari G, Ahlborn J, Rühl M (2021) Role of fungi in fermented foods. In: Zaragoza O, Casadevall A (eds) Encyclopedia of mycology. Elsevier, Amsterdam, pp 590–600
Mahoney RR (1985) Modification of lactose and lactose-containing dairy products with β-galactosidase. In: Fox PF (ed) Developments in dairy chemistry—3. Springer, Dordrecht, pp 69–109
Maicas S (2020) The role of yeasts in fermentation processes. Microorganisms 8:1142. https://doi.org/10.3390/microorganisms8081142
Mapook A, Hyde KD, Hassan K et al (2022) Ten decadal advances in fungal biology leading towards human well-being. Fungal Divers 116:547–614. https://doi.org/10.1007/s13225-022-00510-3
Marini Govigli V, Górriz-Mifsud E, Varela E (2019) Zonal travel cost approaches to assess recreational wild mushroom picking value: trade-offs between online and onsite data collection strategies. For Policy Econ 102:51–65. https://doi.org/10.1016/j.forpol.2019.02.003
Market Data Forecast (2022a) Button mushrooms market. https://www.marketdataforecast.com/market-reports/button-mushrooms-market . accessed 21 Dec 2022
Market Data Forecast (2022b) Rum market. https://www.marketdataforecast.com/market-reports/rum-market . accessed 2 Sep 2022
Market Data Forecast (2022c) Itaconic acid market. https://www.marketdataforecast.com/market-reports/itaconic-acid-market . accessed 31 Oct 2022
Market Data Forecast (2022d) L-ergothioneine market size 2022d: 29.76% CAGR | Current trend, share, competitors and forecast. https://www.marketwatch.com/press-release/l-ergothioneine-market-size-2022d-2976-cagr-current-trend-share-competitors-and-forecast-2022d-10-19 . accessed 12 Nov 2022
Market Data Forecast (2022e) Mycorrhiza-based biofertilizer market. https://www.marketdataforecast.com/market-reports/mycorrhiza-based-biofertilizer-market . accessed 3 Feb 2023
Market Watch (2022a) Penicillin market. https://www.marketwatch.com/press-release/penicillin-market-size-share-trends-massive-growth-is-expected-to-grow-at-a-cagr-of-38-cagr-forecast-by-2028-108-pages-report-2022a-07-21?tesla=y . accessed 21 Jul 2022
Market Watch (2022b) Fusidic acid market. https://www.marketwatch.com/press-release/fusidic-acid-market-size-2022b-spread-across-104-pages-with-technological-advancements-in-report-recent-innovations-value-chain-analysis-till-2028-2022b-07-13#:~:text=219 is 73.27%25.-,The Global Fusidic Acid Market. Accessed 21 Jul 2022
Market Watch (2023a) Mycophenolate Mofetil market and its economic impact on industry with forecast 2028. https://www.marketwatch.com/press-release/mycophenolate-mofetil-market-and-its-economic-impact-on-industry-with-forecast-2028-2023a-03-01 . accessed 12 Mar 2023
Market Watch (2023b) Lentinan market overview 2023b to 2030, future trends and forecast. https://www.marketwatch.com/press-release/lentinan-market-overview-2023b-to-2030-future-trends-and-forecast-ajinomoto-elicityl-nammex-2023b-01-09?mod=search_headline . accessed 19 Jan 2023
Market Watch (2022c) Kojic acid market analysis and insights. https://www.marketwatch.com/press-release/kojic-acid-market-size-2022c-2028-global-industrial-analysis-by-key-companies-profiled-sale-recent-developments-and-forecast-research-report-2022c-10-17#:~:text=Due to the COVID-19,the forecast period 2022c–2028. accessed 12 Nov 2022
Market Watch (2022d) Strobilurin fungicide market size 2023 global gross margin analysis, industry leading players update, development history, business prospect and industry research report 2028. https://www.marketwatch.com/press-release/strobilurin-fungicide-market-size-2023-global-gross-margin-analysis-industry-leading-players-update-development-history-business-prospect-and-industry-research-report-2028-2022b-11-02 . accessed 12 Jan 2023
Markets and Markets (2022a) Lactic acid market. https://www.marketsandmarkets.com/Market-Reports/polylacticacid-387.html?gclid=Cj0KCQjw--2aBhD5ARIsALiRlwDxrAlnCeieNtgEj25leT0adW95t_qWpjTRYnI1ZDUzcsBMxFumT64aApxxEALw_wcB . accessed 29 Oct 2022
Markets and Markets (2022b) Beta glucan market. https://www.marketsandmarkets.com/Market-Reports/beta-glucan-market-5191796.html . accessed 13 Nov 2022
Marquina T, Emery M, Hurley P, Gould RK (2022) The ‘quiet hunt’: the significance of mushroom foraging among Russian-speaking immigrants in New York City. Ecosyst People 18:226–240. https://doi.org/10.1080/26395916.2022.2055148
Martin-Dominguez V, Cabrera PIA, Eidt L et al (2022) Production of fumaric acid by Rhizopus arrhizus NRRL 1526: a simple production medium and the kinetic modelling of the bioprocess. Fermentation 8:64. https://doi.org/10.3390/fermentation8020064
Martin-Lopez B, Montes C, Benayas J (2008) Economic valuation of biodiversity conservation: the meaning of numbers. Conserv Biol 22:624–635. https://doi.org/10.1111/j.1523-1739.2008.00921.x
Martínez AT, Camarero S, Ruiz-Dueñas FJ, Martínez MJ (2018) Biological lignin degradation. In: Beckham G (ed) Lignin Valorization: Emerging Approaches. RSC, Washington DC, pp 199–225
Martinez SJ, Bressani APP, Dias DR et al (2019) Effect of bacterial and yeast starters on the formation of volatile and organic acid compounds in coffee beans and selection of flavors markers precursors during wet fermentation. Front Microbiol 10:1287. https://doi.org/10.3389/fmicb.2019.01287
Martínez de Aragón J, Riera P, Giergiczny M, Colinas C (2011) Value of wild mushroom picking as an environmental service. For Policy Econ 13:419–424. https://doi.org/10.1016/j.forpol.2011.05.003
Matei G, Matei S, Pele M et al (2017) Invertase production by fungi, characterization of enzyme activity and kinetic parameters. Rev Chim 68:2205–2208. https://doi.org/10.37358/RC.17.10.5856
Maximize Market Research (2021) Agarwood oil market: Global industry analysis and forecast (2022–2029). http://www.maximizemarketresearch.com/market-report/global-agarwood-oil-market/107023/ . accessed 9 Jan 2023
May A, Narayanan S, Alcock J et al (2019) Kombucha: a novel model system for cooperation and conflict in a complex multi-species microbial ecosystem. PeerJ 7:e7565. https://doi.org/10.7717/peerj.7565
Menezes AGT, Menezes EGT, Alves JGLF et al (2016) Vodka production from potato ( Solanum tuberosum L. ) using three Saccharomyces cerevisiae isolates. J Inst Brew 122:76–83. https://doi.org/10.1002/jib.302
Metin B (2018) Filamentous fungi in cheese production. In: Budak SO, Akal HC (eds) Microbial cultures and enzymes in dairy technology, 1st edn. IGI Global, Pennsylvania, pp 257–275
Meyer V, Basenko EY, Benz JP et al (2020) Growing a circular economy with fungal biotechnology: a white paper. Fungal Biol Biotechnol 7:5. https://doi.org/10.1186/s40694-020-00095-z
Miller J (2019) Novartis MS drug wins FDA’s blessing amid scrutiny of $88,000 annual price. In: Reuters. https://www.reuters.com/article/us-fda-mayzent-idUSKCN1R8019 . accessed 23 Aug 2022
Miyadera T, Sugimura Y, Hashimoto T et al (1983) Synthesis and in vitro activity of a new carbapenem, RS-533. J Antibiotics 36:1034–1039. https://doi.org/10.7164/antibiotics.36.1034
Mizuno K, Tsujino M, Takada M et al (1974) Studies on bredinin. I. Isolation, characterization and biological properties. J Antibiotics 27:775–782. https://doi.org/10.7164/antibiotics.27.775
Molla AH, Manjurul Haque M, Amdadul Haque M, Ilias GNM (2012) Trichoderma -enriched biofertilizer enhances production and nutritional quality of tomato ( Lycopersicon esculentum Mill.) and minimizes NPK fertilizer use. Agric Res 1:265–272. https://doi.org/10.1007/s40003-012-0025-7
Monteiro de Souza P, De Oliveira e Magalhães P (2010) Application of microbial α-amylase in industry-a review. Braz J Microbiol 41:850–861. https://doi.org/10.1590/S1517-83822010000400004
Monteiro de Souza P, de Bittencourt ML et al (2015) A biotechnology perspective of fungal proteases. Braz J Microbiol 46:337–346. https://doi.org/10.1590/S1517-838246220140359
Mordor Intelligence (2022) Natural food colorants market - growth, trend, Covid-19 impact, and forecast (2022–2027). https://www.mordorintelligence.com/industry-reports/global-natural-food-colorants-market . accessed 3 Nov 2022
Mortimer PE, Karunarathna SC, Li Q et al (2012) Prized edible Asian mushrooms: ecology, conservation and sustainability. Fungal Divers 56:31–47. https://doi.org/10.1007/s13225-012-0196-3
MycoWorks (2023) Grow the future of materials. https://www.mycoworks.com/ . accessed 2 Feb 2023
Nadaroglu H, Polat MS (2022) Microbial extremozymes: novel sources and industrial applications. In: Kuddos M (ed) Microbial extremozymes. Elsevier, Amsterdam, pp 67–88
Naeem M, Manzoor S, Abid M-U-H et al (2022) Fungal proteases as emerging biocatalysts to meet the current challenges and recent developments in biomedical therapies: an updated review. J Fungi 8:109. https://doi.org/10.3390/jof8020109
National Center for Biotechnology Information (2022a) PubChem compound summary for CID 441140, Griseofulvin. https://pubchem.ncbi.nlm.nih.gov/compound/441140 . accessed 20 Aug 2022
National Center for Biotechnology Information (2022b) PubChem compound summary for CID 10690, Gluconic acid. https://pubchem.ncbi.nlm.nih.gov/compound/Gluconic-acid . accessed 21 Sep 2022
NEFFA (2023) The future of fashion manufacturing. https://www.mycotex.nl/ . accessed 2 Feb 2023
Neu HC, Chin NX, Saha G, Labthavikul P (1986) In vitro activity against aerobic and anaerobic gram-positive and gram-negative bacteria and beta-lactamase stability of RS-533, a novel carbapenem. Antimicrob Agents Chemother 30:828–834. https://doi.org/10.1128/AAC.30.6.828
Newman DJ, Cragg GM (2020) Natural products as sources of new drugs over the nearly four decades from 01/1981 to 09/2019. J Nat Prod 83:770–803. https://doi.org/10.1021/acs.jnatprod.9b01285
Newton GG, Abraham EP (1955) Cephalosporin C, a new antibiotic containing sulphur and d -alpha-aminoadipic acid. Nature 175:548. https://doi.org/10.1038/175548a0
Nguyen T, Karl M, Santini A (2017) Red yeast rice. Foods 6:19. https://doi.org/10.3390/foods6030019
Niego AG, Rapior S, Thongklang N et al (2021) Macrofungi as a nutraceutical source: promising bioactive compounds and market value. J Fungi 7:397. https://doi.org/10.3390/jof7050397
Niego AGT, Rapior S, Thongklang N et al (2023) Reviewing the contributions of macrofungi to forest ecosystem processes and services. Fungal Biol Rev 44:100294. https://doi.org/10.1016/j.fbr.2022.11.002
Nigam PS, Singh A (2014) Single cell protein | Mycelial fungi. In: Batt CA, Tortorello ML (eds) Encyclopedia of Food microbiology, 2nd edn. Elsevier, Amsterdam, pp 415–424
Nofiani R, de Mattos-Shipley K, Lebe KE et al (2018) Strobilurin biosynthesis in basidiomycete fungi. Nat Commun 9:3940. https://doi.org/10.1038/s41467-018-06202-4
OEC (2022a) Blue Cheeses. https://oec.world/en/profile/hs/cheese-blue-veined . accessed 27 Aug 2022
OEC (2022b) Baked goods. https://oec.world/en/profile/hs/baked-goods . accessed 27 Aug 2022
OEC (2022c) Cheese. https://oec.world/en/profile/hs/cheese . accessed 27 Aug 2022
OEC (2022d) Coffee. https://oec.world/en/profile/hs/coffee#exporters-importers . accessed 5 Sep 2022
Oxford AE, Raistrick H, Simonart P (1939) Studies in the biochemistry of micro-organisms: Griseofulvin, C(17)H(17)O(6)Cl, a metabolic product of Penicillium griseo-fulvum Dierckx. Biochem J 33:240–248. https://doi.org/10.1042/bj0330240
Pan X, Liu H, Liu J et al (2016) Omics-based approaches reveal phospholipids remodeling of Rhizopus oryzae responding to furfural stress for fumaric acid-production from xylose. Bioresour Technol 222:24–32. https://doi.org/10.1016/j.biortech.2016.09.101
Pandey A, Höfer R, Taherzadeh M et al (2015) Industrial biorefineries & white biotechnology. Elsevier, Amsterdam
Pandey A, Nigam P, Soccol CR et al (2000) Advances in microbial amylases. Biotechnol Appl Biochem 31:135. https://doi.org/10.1042/BA19990073
Papp-Wallace KM, Endimiani A, Taracila MA, Bonomo RA (2011) Carbapenems: past, present, and future. Antimicrob Agents Chemother 55:4943–4960. https://doi.org/10.1128/AAC.00296-11
Paterson RRM (2008) Cordyceps – A traditional Chinese medicine and another fungal therapeutic biofactory? Phytochemistry 69:1469–1495. https://doi.org/10.1016/j.phytochem.2008.01.027
Paterson RRM (2006) Ganoderma –a therapeutic fungal biofactory. Phytochemistry 67:1985–2001. https://doi.org/10.1016/j.phytochem.2006.07.004
Paukner S, Riedl R (2017) Pleuromutilins: potent drugs for resistant bugs-mode of action and resistance. Cold Spring Harb Perspect Med 7:a027110. https://doi.org/10.1101/cshperspect.a027110
Pauley M, Maskell D (2017) Mini-review: The role of Saccharomyces cerevisiae in the production of gin and vodka. Beverages 3:13. https://doi.org/10.3390/beverages3010013
Pedersen TR, Tobert JA (2004) Simvastatin: a review. Expert Opin Pharmacother 5:2583–2596. https://doi.org/10.1517/14656566.5.12.2583
Peláez F, Cabello A, Platas G et al (2000) The discovery of enfumafungin, a novel antifungal compound produced by an endophytic Hormonema species biological activity and taxonomy of the producing organisms. Syst Appl Microbiol 23:333–343. https://doi.org/10.1016/S0723-2020(00)80062-4
Pérez-Chávez AM, Mayer L, Albertó E (2019) Mushroom cultivation and biogas production: a sustainable reuse of organic resources. Energy Sustain Dev 50:50–60
Persistence Market Research (2022) Red yeast rice market. https://www.persistencemarketresearch.com/market-research/red-yeast-rice-market.asp . accessed 19 Jan 2023
Phasha V, Senabe J, Ndzotoyi P et al (2022) Review on the use of kojic acid—a skin-lightening ingredient. Cosmetics 9:64. https://doi.org/10.3390/cosmetics9030064
Polaris Market Research (2022) Carbapenem market share, size, trends, industry analysis report, by product type (Meropenem, Doripenem, Imipenem, Tebipenem, and others); by sales channel; by region; segment forecast, 2022–2030. https://www.polarismarketresearch.com/industry-analysis/carbapenem-market . accessed 3 Feb 2023
Polaris Market Research (2021) Kombucha market share, trends, size, industry analysis report, by product (organic, inorganic), by type (natural, flavored), by microbial, by distribution channel, by region; Segment forecast, 2022–2030. https://www.polarismarketresearch.com/industry-analysis/kombucha-market . accessed 6 May 2023
Poorniammal R, Prabhu S, Dufossé L, Kannan J (2021) Safety evaluation of fungal pigments for food applications. J Fungi 7:692. https://doi.org/10.3390/jof7090692
Prenafeta-Boldú FX, de Hoog GS, Summerbell RC (2019) Fungal communities in hydrocarbon degradation. In: McGenity TJ (ed) Microbial communities utilizing hydrocarbons and lipids: members, metagenomics and ecophysiology. Springer, Cham, pp 307–342
Pritchard PE (1992) Studies on the bread-improving mechanism of fungal alpha-amylase. J Biol Educ 26:12–18. https://doi.org/10.1080/00219266.1992.9655237
PS Market Research (2022) Industrial enzymes market research report. https://www.psmarketresearch.com/market-analysis/industrial-enzymes-market . accessed 31 Oct 2022
Raghoonundon B, Gonkhom D, Phonemany M et al (2021) Nutritional content, nutraceutical properties, cultivation methods and economical importance of Lentinula : a review. Fungal Biotec 1:88–100. https://doi.org/10.5943/FunBiotec/1/2/6
Rakhee MJ, Yadav RB et al (2021) Novel formulation development from Ophiocordyceps sinensis (Berk) for management of high-altitude maladies. 3 Biotech 11:9. https://doi.org/10.1007/s13205-020-02536-3
Rathore H, Prasad S, Sharma S (2017) Mushroom nutraceuticals for improved nutrition and better human health: a review. PharmaNutrition 5:35–46. https://doi.org/10.1016/j.phanu.2017.02.001
Refinitiv (2022) Carbon trading: exponential growth on record high. https://www.refinitiv.com/perspectives/market-insights/carbon-trading-exponential-growth-on-record-high/ . accessed 11 Jan 2023
Reportlinker (2022) Global fumaric acid industry. https://www.reportlinker.com/p05817775/Global-Fumaric-Acid-Industry.html?utm_source=GNW . accessed 31 Oct 2022
Research and Markets (2022a) Coffee market - growth, trends, COVID-19 impact, and forecasts (2022a - 2027). https://www.researchandmarkets.com/reports/5165416/coffee-market-growth-trends-covid-19-impact?utm_source=BW&utm_medium=PressRelease&utm_code=zj95v9&utm_campaign=1671722+-+%24102+Billion+Global+Coffee+Market+Growth%2C+Trends%2C+COVID-19+Impact%2C+and+Fore . accessed 5 Sep 2022
Research and Markets (2022b) Biopesticides market by type. https://www.researchandmarkets.com/reports/5116567/biopesticides-market-by-type-bioinsecticides?utm_source=GNOM&utm_medium=PressRelease&utm_code=g36rbx&utm_campaign=1736666+-+Global+Biopesticides+Market+Report+2022b-2027%3A+Pests+Developing+Resistance+to+C . accessed 12 Jan 2023
Research and Markets (2022c) Global mycelium market analysis and forecast, 2020–2021 & 2022c-2026 - adoption of mycelium in pharmaceutical and skin care industry. https://www.researchandmarkets.com/reports/5559925/mycelium-market-a-global-and-regional-analysis?utm_source=GNOM&utm_medium=PressRelease&utm_code=j2k7m4&utm_campaign=1685003+-+Global+Mycelium+Market+Analysis+and+Forecast%2C+2020-2021+%26+2022c-2026+-+Adop . accessed 21 Jun 2022
Rezac S, Kok CR, Heermann M, Hutkins R (2018) Fermented foods as a dietary source of live organisms. Front Microbiol 9:1785. https://doi.org/10.3389/fmicb.2018.01785
Rodríguez-Sáiz M, de la Fuente JL, Barredo JL (2010) Xanthophyllomyces dendrorhous for the industrial production of astaxanthin. Appl Microbiol Biotechnol 88:645–658. https://doi.org/10.1007/s00253-010-2814-x
Ropars J, Didiot E, Rodríguez de la Vega RC et al (2020) Domestication of the emblematic white cheese-making fungus Penicillium camemberti and its diversification into two varieties. Curr Biol 30:4441–4453. https://doi.org/10.1016/j.cub.2020.08.082
Rosa LH, Machado KMG, Jacob CC et al (2003) Screening of Brazilian basidiomycetes for antimicrobial activity. Mem Inst Oswaldo Cruz 98:967–974. https://doi.org/10.1590/S0074-02762003000700019
Rosa LH, Cota BB, Machado KMG et al (2005) Antifungal and other biological activities from Oudemansiella canarii (Basidiomycota). World J Microbiol Biotechnol 21:983–987. https://doi.org/10.1007/s11274-004-7553-7
Royse DJ, Baars J, Tan Q (2017) Current overview of mushroom production in the world. In: Diego CZ, Pardo-Giménez A (eds) Edible and medicinal mushrooms. Wiley, Chichester, pp 5–13
Rüegger A, Kuhn M, Lichti H et al (1976) Cyclosporin A, a peptide metabolite from Trichoderma polysporum (Link ex Pers.) Rifai, with a remarkable immunosuppressive activity. Helv Chim Acta 59:1075–1092. https://doi.org/10.1002/hlca.19760590412
Ruta LL, Farcasanu IC (2021) Coffee and yeasts: from flavor to biotechnology. Fermentation 7:9. https://doi.org/10.3390/fermentation7010009
Saeedi M, Eslamifar M, Khezri K (2019) Kojic acid applications in cosmetic and pharmaceutical preparations. Biomed Pharmacother 110:582–593. https://doi.org/10.1016/j.biopha.2018.12.006
Saini S, Sharma KK (2021) Fungal lignocellulolytic enzymes and lignocellulose: a critical review on their contribution to multiproduct biorefinery and global biofuel research. Int J Biol Macromol 193:2304–2319. https://doi.org/10.1016/j.ijbiomac.2021.11.063
San-Juan-Rodriguez A, Good CB, Heyman RA et al (2019) Trends in prices, market share, and spending on self-administered disease-modifying therapies for multiple sclerosis in medicare part D. JAMA Neurol 76:1386. https://doi.org/10.1001/jamaneurol.2019.2711
Sandargo B, Chepkirui C, Cheng T et al (2019) Biological and chemical diversity go hand in hand: Basidiomycota as source of new pharmaceuticals and agrochemicals. Biotechnol Adv 37:107344. https://doi.org/10.1016/j.biotechadv.2019.01.011
Sandmann G (2022) Carotenoids and their biosynthesis in fungi. Molecules 27:1431. https://doi.org/10.3390/molecules27041431
Sandoval-Lozano CJ, Caballero-Torres D, López-Giraldo LJ (2022) Screening wild yeast isolated from cocoa bean fermentation using volatile compounds profile. Molecules 27:902. https://doi.org/10.3390/molecules27030902
Saqib S, Akram A, Halim SA, Tassaduq R (2017) Sources of β-galactosidase and its applications in food industry. 3 Biotech 7:79. https://doi.org/10.1007/s13205-017-0645-5
Sasaki T, Takagi M, Yaguchi T et al (1992) A new anthelmintic cyclodepsipeptide, PF1022A. J Antibiotics 45:692–697. https://doi.org/10.7164/antibiotics.45.692
Sawistowska-Schröder ET, Kerridge D, Perry H (1984) Echinocandin inhibition of 1,3-beta- d -glucan synthase from Candida albicans . FEBS Lett 173:134–138. https://doi.org/10.1016/0014-5793(84)81032-7
Scherkenbeck J, Jeschke P, Harder A (2002) PF1022A and related cyclodepsipeptides-a novel class of anthelmintics. Curr Top Med Chem 2:759–777. https://doi.org/10.2174/1568026023393624
Schmidt L, Skvortsova V, Kullen C et al (2017) How context alters value: the brain’s valuation and affective regulation system link price cues to experienced taste pleasantness. Sci Rep 7:8098. https://doi.org/10.1038/s41598-017-08080-0
Schulp CJE, Thuiller W, Verburg PH (2014) Wild food in Europe: a synthesis of knowledge and data of terrestrial wild food as an ecosystem service. Ecol Econ 105:292–305. https://doi.org/10.1016/j.ecolecon.2014.06.018
Schwartz RE, Giacobbe RA, Bland JA, Monaghan RL (1989) L-671,329, a new antifungal agent. I. Fermentation and isolation. J Antibiotics 42:163–167. https://doi.org/10.7164/antibiotics.42.163
Scynexis (2022) SCYNEXIS reports fourth quarter and full year 2021 financial results and provides corporate update. https://www.scynexis.com/news-media/press-releases/detail/278/scynexis-reports-fourth-quarter-and-full-year-2021 . accessed 23 Aug 2022
Sebastiana M, da Silva AB, Matos AR et al (2018) Ectomycorrhizal inoculation with Pisolithus tinctorius reduces stress induced by drought in cork oak. Mycorrhiza 28:247–258. https://doi.org/10.1007/s00572-018-0823-2
Seyis I, Aksoz N (2004) Production of lactase by Trichoderma sp. Food Technol Biotechnol 42:121–124
Sharma N, Sahota P, Singh M (2021) Organic acid production from agricultural waste. In: Kashyap BK, Solanki MK, Kamboj DV, Pandey AK (eds) Waste to energy: prospects and applications. Springer, Singapore, pp 415–438
Show PL, Oladele KO, Siew QY et al (2015) Overview of citric acid production from Aspergillus niger . Front Life Sci 8:271–283. https://doi.org/10.1080/21553769.2015.1033653
Shrestha UB (2012) Asian medicine: a fungus in decline. Nature 482:35. https://doi.org/10.1038/482035b
Silverman J, Cao H, Cobb K (2020) Development of mushroom mycelium composites for footwear products. Cloth Text Res J 38:119–133. https://doi.org/10.1177/0887302X19890006
Singh AK, Mukhopadhyay M (2012) Overview of fungal lipase: a review. Appl Biochem Biotechnol 166:486–520. https://doi.org/10.1007/s12010-011-9444-3
Singh RS, Chauhan K, Singh RP (2017) Enzymatic approaches for the synthesis of high fructose syrup. In: Gahlawat S, Salar R, Siwach P, Duhan J, Kumar S, Kaur P (eds) Plant biotechnology: recent advancements and developments. Springer, Singapore, pp 189–211
Sjamsuridzal W, Khasanah M, Febriani R et al (2021) The effect of the use of commercial tempeh starter on the diversity of Rhizopus tempeh in Indonesia. Sci Rep 11:23932. https://doi.org/10.1038/s41598-021-03308-6
Ślusarczyk J, Adamska E, Czerwik-Marcinkowska J (2021) Fungi and algae as sources of medicinal and other biologically active compounds: a review. Nutrients 13:3178. https://doi.org/10.3390/nu13093178
Solieri L, Giudici P (2008) Yeasts associated to traditional balsamic vinegar: ecological and technological features. Int J Food Microbiol 125:36–45. https://doi.org/10.1016/j.ijfoodmicro.2007.06.022
Soudzilovskaia NA, Vaessen S, Barcelo M et al (2020) FungalRoot: global online database of plant mycorrhizal associations. New Phytol 227:955–966. https://doi.org/10.1111/nph.16569
Soudzilovskaia NA, van Bodegom PM, Terrer C et al (2019) Global mycorrhizal plant distribution linked to terrestrial carbon stocks. Nat Commun 10:5077. https://doi.org/10.1038/s41467-019-13019-2
Special Chem (2022) Parnika mushroom. https://cosmetics.specialchem.com/product/i-parnika-parnika-mushroom . accessed 2 Dec 2022
Statista (2022a) Global biofuels market size 2020–2030. https://www.statista.com/statistics/217179/global-biofuels-market-size/#:~:text=The global biofuels market was,to 201.2 billion U.S. dollars. accessed 20 Jun 2022
Statista (2022b) Global gross domestic product (GDP) at current prices from 1985 to 2027(in billion U.S. dollars). https://www.statista.com/statistics/268750/global-gross-domestic-product-gdp/#:~:text=The statistic shows global gross,trillion lower than in 2019. accessed 10 Mar 2023
Steiger MG, Blumhoff ML, Mattanovich D, Sauer M (2013) Biochemistry of microbial itaconic acid production. Front Microbiol 4:23. https://doi.org/10.3389/fmicb.2013.00023
Steinberg D (2006) Thematic review series: the pathogenesis of atherosclerosis. An interpretive history of the cholesterol controversy, part V: the discovery of the statins and the end of the controversy. J Lipid Res 47:1339–1351. https://doi.org/10.1194/jlr.R600009-JLR200
Stelzer L, Hoberg F, Bach V et al (2021) Life cycle assessment of fungal-based composite bricks. Sustainability 13:11573. https://doi.org/10.3390/su132111573
Stoffel F, de Santana W, O, Fontana RC, Camassola M, (2021) Use of Pleurotus albidus mycoprotein flour to produce cookies: Evaluation of nutritional enrichment and biological activity. Innov Food Sci Emerg Technol 68:102642. https://doi.org/10.1016/j.ifset.2021.102642
Straits Research (2021) Agarwood chip market. https://straitsresearch.com/report/agarwood-chip-market/ . accessed 14 Jan 2023
Sudha GC, Aggarwal S (2016) Dyeing wet blue goat nappa skin with a microbial colorant obtained from Penicillium minioluteum . J Clean Prod 127:585–590. https://doi.org/10.1016/j.jclepro.2016.03.043
Suhaimi H, Dailin DJ, Malek RA et al (2021) Fungal pectinases: production and applications in food industries. In: Dai X, Sharma M, Chen J (eds) Fungi in sustainable food production. Springer, Cham, pp 85–116
Sun W, Tajvidi M, Hunt CG et al (2019) Fully bio-based hybrid composites made of wood, fungal mycelium and cellulose nanofibrils. Sci Rep 9:3766. https://doi.org/10.1038/s41598-019-40442-8
Sunagawa M, Matsumura H, Inoue T et al (1990) A novel carbapenem antibiotic, SM-7338 structure-activity relationships. J Antibiotics 43:519–532. https://doi.org/10.7164/antibiotics.43.519
Sundelof JG, Hajdu R, Gill CJ et al (1997) Pharmacokinetics of L-749,345, a long-acting carbapenem antibiotic, in primates. Antimicrob Agents Chemother 41:1743–1748. https://doi.org/10.1128/AAC.41.8.1743
Survase SA, Kagliwal LD, Annapure US, Singhal RS (2011) Cyclosporin A–a review on fermentative production, downstream processing and pharmacological applications. Biotechnol Adv 29:418–435. https://doi.org/10.1016/j.biotechadv.2011.03.004
Sydor M, Bonenberg A, Doczekalska B, Cofta G (2021) Mycelium-based composites in art, architecture, and interior design: a review. Polymers (basel) 14:145. https://doi.org/10.3390/polym14010145
Takala T, Lehtinen A, Hujala T et al (2021) Forest owners as political actors. Environ Sci Policy 126:22–30. https://doi.org/10.1016/j.envsci.2021.09.009
Talukdar D, Jasrotia T, Sharma R et al (2020) Evaluation of novel indigenous fungal consortium for enhanced bioremediation of heavy metals from contaminated sites. Environ Technol Innov 20:101050. https://doi.org/10.1016/j.eti.2020.101050
Tamang JP, Cotter PD, Endo A et al (2020) Fermented foods in a global age: East meets West. Compr Rev Food Sci Food Saf 19:184–217. https://doi.org/10.1111/1541-4337.12520
Tanret C (1909) Sur une base nouvelle retiree du seigle ergote, l’ergothioneine. Comptes Rend Acad Sci 149:222–224
Teleky B-E, Vodnar D (2019) Biomass-derived production of itaconic acid as a building block in specialty polymers. Polymers (basel) 11:1035. https://doi.org/10.3390/polym11061035
Terada M, Hayashi K, Mizunuma T (1981) Enzyme productivity and identification of species of Koji-molds for soy sauce distributed in Japan. J Soy Sauce Res Technol 7:158–165
Thakur MP (2020) Advances in mushroom production: key to food, nutritional and employment security: a review. Indian Phytopathol 73:377–395
The Brainy Insights (2022) Shiitake mushroom market size by product type. https://www.thebrainyinsights.com/report/shiitake-mushroom-market-12716#:~:text=As per The Brainy Insights,USD 4.7 billion by 2030. accessed 20 Dec 2022
The Business Research Company (2022) Tempeh global market report 2022. https://www.thebusinessresearchcompany.com/report/tempeh-global-market-report#:~:text=This tempeh market research report,(CAGR) of 7.8%25. Accessed 31 Oct 2022
The Business Research Company (2023) Cheese global market report 2023. https://www.thebusinessresearchcompany.com/report/cheese-global-market-report . accessed 25 Jan 2023
The Spirit Business (2022g) Tequila sales to reach $24.19 billion by 2031. https://www.thespiritsbusiness.com/2022g/08/tequila-sales-to-reach-24-19-billion-by-2031/ . accessed 5 Sep 2022
Thomas K, Proschmann U, Ziemssen T (2017) Fingolimod hydrochloride for the treatment of relapsing remitting multiple sclerosis. Expert Opin Pharmacother 18:1649–1660. https://doi.org/10.1080/14656566.2017.1373093
Tibuhwa DD (2013) Wild mushroom- an underutilized healthy food resource and income generator: experience from Tanzania rural areas. J Ethnobiol Ethnomed 9:49. https://doi.org/10.1186/1746-4269-9-49
Tonkinson B (2022h) How to make vinegar. https://ice.edu/blog/making-vinegars#:~:text=The yeast consumes the natural,to take place%2C acetic fermentation. accessed 5 Sep 2022
Tridge (2022a) Hericium. https://www.tridge.com/intelligences/hericium-mushrooms/PL . accessed 30 Aug 2022
Tridge (2022b) Lion’s mane mushroom. https://www.tridge.com/intelligences/lions-mane-mushroom . accessed 21 Dec 2022
Tsuji M, Ishii Y, Ohno A et al (1998) In vitro and in vivo antibacterial activities of S-4661, a new carbapenem. Antimicrob Agents Chemother 42:94–99. https://doi.org/10.1128/AAC.42.1.94
Tulpule V, Brown S, Lim J, et al (1998) An economic assessment of the Kyoto Protocol using the global trade and environment model. In: The Economic Modelling of Climate Change: Background Analysis for the Kyoto Protocol OECD Workshop. Paris
Ubukata K, Hikida M, Yoshida M et al (1990) In vitro activity of LJC10,627, a new carbapenem antibiotic with high stability to dehydropeptidase I. Antimicrob Agents Chemother 34:994–1000. https://doi.org/10.1128/AAC.34.6.994
UNData (2022i) Mushroom and truffles. http://data.un.org/Data.aspx?d=FAO&f=itemCode:449 . accessed 13 May 2022
Usman M, Murtaza G, Ditta A (2021) Nutritional, medicinal, and cosmetic value of bioactive compounds in button mushroom ( Agaricus bisporus ): a review. Appl Sci 11:5943. https://doi.org/10.3390/app11135943
Valavanidis A (2018) Concept and practice of the circular economy. Sci Rev 143:2–5
Valverde ME, Hernández-Pérez T, Paredes-López O (2015) Edible mushrooms: improving human health and promoting quality life. Int J Microbiol 2015:376387. https://doi.org/10.1155/2015/376387
Varghese R, Dalvi YB, Lamrood PY et al (2019) Historical and current perspectives on therapeutic potential of higher basidiomycetes: an overview. 3 Biotech 9:362. https://doi.org/10.1007/s13205-019-1886-2
Venil CK, Velmurugan P, Dufossé L et al (2020) Fungal pigments: potential coloring compounds for wide ranging applications in textile dyeing. J Fungi 6:68. https://doi.org/10.3390/jof6020068
Verified Market Research (2022a) Cyclosporine market size and forecast. https://www.verifiedmarketresearch.com/product/cyclosporine-market/ . accessed 21 Aug 2022
Verified Market Research (2022b) Soy sauce market size and forecast. https://www.verifiedmarketresearch.com/product/soy-sauce-market/ . accessed 31 Oct 2022
Verma ML, Thakur M, Randhawa JS et al (2020) Biotechnological applications of fungal enzymes with special reference to bioremediation. In: Gothandam KM, Ranjan S, Dasgupta N, Lichtfouse E (eds) Environmental biotechnology, vol 2. Springer, Cham, pp 221–247
Vieira GAL, Magrini MJ, Bonugli-Santos RC et al (2018) Polycyclic aromatic hydrocarbons degradation by marine-derived basidiomycetes: optimization of the degradation process. Braz J Microbiol 49:749–756. https://doi.org/10.1016/j.bjm.2018.04.007
Vilela A (2019) The importance of yeasts on fermentation quality and human health-promoting compounds. Fermentation 5:46. https://doi.org/10.3390/fermentation5020046
Vishwanatha KS, Rao AGA, Singh SA (2010) Acid protease production by solid-state fermentation using Aspergillus oryzae MTCC 5341: optimization of process parameters. J Ind Microbiol Biotechnol 37:129–138. https://doi.org/10.1007/s10295-009-0654-4
Volpi C, Orabona C, Macchiarulo A et al (2019) Preclinical discovery and development of fingolimod for the treatment of multiple sclerosis. Expert Opin Drug Discov 14:1199–1212. https://doi.org/10.1080/17460441.2019.1646244
Walbank J (2022) Can yeast processing improve the quality of coffee?. https://mtpak.coffee/2022/04/yeast-processing-can-it-improve-quality-of-coffee/
Walker G, Stewart G (2016) Saccharomyces cerevisiae in the production of fermented beverages. Beverages 2:30. https://doi.org/10.3390/beverages2040030
Wang C, Sun H, Li J et al (2009) Enzyme activities during degradation of polycyclic aromatic hydrocarbons by white rot fungus Phanerochaete chrysosporium in soils. Chemosphere 77:733–738. https://doi.org/10.1016/j.chemosphere.2009.08.028
Wang J, Zhou Z, Dan D, Hu G (2020) Physicochemical properties and bioactivities of Lentinula edodes polysaccharides at different development stages. Int J Biol Macromol 150:573–577. https://doi.org/10.1016/j.ijbiomac.2020.02.099
Wierckx N, Agrimi G, Lübeck PS et al (2020) Metabolic specialization in itaconic acid production: a tale of two fungi. Curr Opin Biotechnol 62:153–159. https://doi.org/10.1016/j.copbio.2019.09.014
Willson J, Amliwala K, Harder A et al (2003) The effect of the anthelmintic emodepside at the neuromuscular junction of the parasitic nematode Ascaris suum . Parasitology 126:79–86. https://doi.org/10.1017/s0031182002002639
Wilson LA (1995) Practical handbook of soybean processing and utilization. Elsevier, Amsterdam
Wisitrassameewong K, Karunarathna S, Thongklang N et al (2012) Agaricus subrufescens : a review. Saudi J Biol Sci 19:131–146. https://doi.org/10.1016/j.sjbs.2012.01.003
Wittstein K, Cordsmeier A, Lambert C et al (2020) Identification of Rosellinia species as producers of cyclodepsipeptide PF1022 A and resurrection of the genus Dematophora as inferred from polythetic taxonomy. Stud Mycol 96:1–16. https://doi.org/10.1016/j.simyco.2020.01.001
World Economic Forum (2022) Why mushroom mycelium could be your next house, handbag, or ‘hamburger.’ https://www.weforum.org/agenda/2020/12/mycelium-mushroom-sustainable-packaging-fashion-meat/ . accessed 20 Jun 2022
World Population Review (2022) Spain population 2022. https://worldpopulationreview.com/countries/spain-population . accessed 19 Jun 2022
Wright AJ (1999) The penicillins. Mayo Clin Proc 74:290–307. https://doi.org/10.4065/74.3.290
Wu Y, Choi M-H, Li J et al (2016) Mushroom cosmetics: the present and future. Cosmetics 3:22. https://doi.org/10.3390/cosmetics3030022
Yadav P, Chauhan AK, Singh RB et al (2022) Organic acids: microbial sources, production, and applications. In: Singh RB, Watanabe S, Isaza AA (eds) Functional foods and nutraceuticals in metabolic and non-communicable diseases. Elsevier, Amsterdam, pp 325–337
Yang ST, Zhang K, Zhang B, Huang H (2011) 3.16 - Fumaric acid. In: Moo-Young M (ed) Comprehensive biotechnology, 2nd edn. Academic Press, Burlington, pp 163–177
Yokotsuka T, Sasaki M (1998) Fermented protein foods in the Orient: shoyu and miso in Japan. In: Wood BJB (ed) Microbiology of fermented foods. Springer, Boston, pp 351–415
Zaki O, Weekers F, Thonart P et al (2020) Limiting factors of mycopesticide development. Biol Control 144:104220. https://doi.org/10.1016/j.biocontrol.2020.104220
Zeb M, Lee CH (2021) Medicinal properties and bioactive compounds from wild mushrooms native to North America. Molecules 26:251. https://doi.org/10.3390/molecules26020251
Zha LS, Huang SK, Xiao YP et al (2018) An evaluation of common Cordyceps (Ascomycetes) species found in Chinese markets. Int J Med Mushrooms 20:1149–1162. https://doi.org/10.1615/IntJMedMushrooms.2018027330
Zhang N, Zhao X-H (2010) Study of Mucor spp. in semi-hard cheese ripening. J Food Sci Technol 47:613–619. https://doi.org/10.1007/s13197-010-0108-z
Zhang ZY, Jin B, Kelly JM (2007) Production of lactic acid from renewable materials by Rhizopus fungi. Biochem Eng J 35:251–263. https://doi.org/10.1016/j.bej.2007.01.028
Zhang N, Guo Q-Q, Zhao X-H (2012) A brief investigation on the proteolysis and textural modification of a semi-hard cheese ripened by Mucor spp. Biotechnology 12:54–60. https://doi.org/10.3923/biotech.2013.54.60
Zhang HW, Lin ZX, Tung YS et al (2014) Cordyceps sinensis (a traditional Chinese medicine) for treating chronic kidney disease. Cochrane Database Syst Rev 2014:CD008353. https://doi.org/10.1002/14651858.CD008353.pub2
Zhang J, Chen Q, Huang C, Gao W (2015) History, current situation and trend of edible mushroom industry development. Mycosystema 34:524–540. https://doi.org/10.13346/j.mycosystema.150076-en
Zhang Y, He S, Simpson BK (2018) Enzymes in food bioprocessing—novel food enzymes, applications, and related techniques. Curr Opin Food Sci 19:30–35. https://doi.org/10.1016/j.cofs.2017.12.007
Zhen X, Wang Y, Liu D (2020) Bio-butanol as a new generation of clean alternative fuel for SI (spark ignition) and CI (compression ignition) engines. Renew Energy 147:2494–2521. https://doi.org/10.1016/j.renene.2019.10.119
Zhen J, Wang J, Li L et al (2022) Biodegradation of PAHs by Trametes hirsuta zlh237 and effect of bioaugmentation on PAHs-contaminated soil. Polish J Environ Stud 31:2473–2484. https://doi.org/10.15244/pjoes/143010
Zhou QZK, Chen XD (2001) Effects of temperature and pH on the catalytic activity of the immobilized β-galactosidase from Kluyveromyces lactis . Biochem Eng J 9:33–40. https://doi.org/10.1016/S1369-703X(01)00118-8
Zhu J-S, Halpern GM, Jones K (1998) The scientific rediscovery of an ancient Chinese herbal medicine: Cordyceps sinensis Part I. J Altern Complement Med 4:289–303. https://doi.org/10.1089/acm.1998.4.3-289
Zi Y, Jiang B, He C, Liu L (2020) Lentinan inhibits oxidative stress and inflammatory cytokine production induced by benzo(a)pyrene in human keratinocytes. J Cosmet Dermatol 19:502–507. https://doi.org/10.1111/jocd.13005
Zotti M, Persiani AM, Ambrosio E et al (2013) Macrofungi as ecosystem resources: conservation versus exploitation. Plant Biosyst 147:219–225. https://doi.org/10.1080/11263504.2012.753133
Download references
The authors also acknowledge Dr. Eric Boa for sharing his studies and publications. We thank Dr. Olivier Raspé for helping in the initial conceptualization of the paper.
Open Access funding enabled and organized by Projekt DEAL. This work was in part supported by the Thailand Science Research and Innovation grant “Macrofungi diversity research from the Lancang-Mekong Watershed and surrounding areas” (Grant No. DBG6280009) and the National Research Council of Thailand (NRCT) grant “Total fungal diversity in a given forest area with implications towards species numbers, chemical diversity and biotechnology” (Grant No. N42A650547. We thank the Mushroom Research Foundation, Chiang Rai, Thailand for supporting the Ph.D studies of A.G. Niego. Niego would like to acknowledge the Mae Fah Luang University Thesis/Dissertation Support Grant (Reference No. Oh 7702(6)/49). EC-G was supported by the HZI POF IV Cooperativity and Creativity Project Call and CL was funded by a PhD stipend of the Life Science Foundation, Munich.
Author information
Authors and affiliations.
School of Science, Mae Fah Luang University, Chiang Rai, Thailand
Allen Grace T. Niego, Naritsada Thongklang, Arttapon Walker & Kevin D. Hyde
Center of Excellence in Fungal Research, Mae Fah Luang University, Chiang Rai, Thailand
Iloilo Science and Technology University, La Paz, 5000, Iloilo, Philippines
Allen Grace T. Niego
Department Microbial Drugs, Helmholtz Centre for Infection Research, Inhoffenstrasse 7, 38124, Brunswick, Germany
Christopher Lambert, Miriam Grosse, Hedda Schrey, Esteban Charria-Girón & Marc Stadler
Institute of Microbiology, Technische Universität Braunschweig, Spielmannstraße 7, 38106, Brunswick, Germany
Key Laboratory for Plant Diversity and Biogeography of East Asia, Kunming Institute of Botany, Chinese Academy of Sciences, Kunming, 650201, Yunnan, People’s Republic of China
Peter Mortimer
Laboratory of Botany, Phytochemistry and Mycology, Faculty of Pharmacy, CS 14491, 15 Avenue Charles Flahault, 34093, Montpellier Cedex 5, France
Sylvie Rapior
CEFE, CNRS, Univ Montpellier, EPHE, IRD, CS 14491, 15 Avenue Charles Flahault, 34093, Montpellier Cedex 5, France
Department of Biology, Faculty of Science, Chiang Mai University, Chiang Mai, 50200, Thailand
Kevin D. Hyde
Institute for Plant Health, Zhongkai University of Agriculture and Engineering, Haizhu District, Guangzhou, 510225, People’s Republic of China
You can also search for this author in PubMed Google Scholar
Contributions
The first draft of the manuscript was written by AGN and all authors commented on previous versions of the manuscript. All authors read and approved the final manuscript.
Corresponding authors
Correspondence to Kevin D. Hyde or Marc Stadler .
Ethics declarations
Competing interests.
The authors declare no competing interests.
Additional information
Handling Editor: R. Jeewon.
Rights and permissions
Open Access This article is licensed under a Creative Commons Attribution 4.0 International License, which permits use, sharing, adaptation, distribution and reproduction in any medium or format, as long as you give appropriate credit to the original author(s) and the source, provide a link to the Creative Commons licence, and indicate if changes were made. The images or other third party material in this article are included in the article's Creative Commons licence, unless indicated otherwise in a credit line to the material. If material is not included in the article's Creative Commons licence and your intended use is not permitted by statutory regulation or exceeds the permitted use, you will need to obtain permission directly from the copyright holder. To view a copy of this licence, visit http://creativecommons.org/licenses/by/4.0/ .
Reprints and permissions
About this article
Niego, A.G.T., Lambert, C., Mortimer, P. et al. The contribution of fungi to the global economy. Fungal Diversity 121 , 95–137 (2023). https://doi.org/10.1007/s13225-023-00520-9
Download citation
Received : 15 March 2023
Accepted : 29 May 2023
Published : 12 July 2023
Issue Date : July 2023
DOI : https://doi.org/10.1007/s13225-023-00520-9
Share this article
Anyone you share the following link with will be able to read this content:
Sorry, a shareable link is not currently available for this article.
Provided by the Springer Nature SharedIt content-sharing initiative
- Fungi-based food
- Medicinal mushrooms
- Market value
- Environmental biotechnology
- Find a journal
- Publish with us
- Track your research
Thank you for visiting nature.com. You are using a browser version with limited support for CSS. To obtain the best experience, we recommend you use a more up to date browser (or turn off compatibility mode in Internet Explorer). In the meantime, to ensure continued support, we are displaying the site without styles and JavaScript.
- View all journals
- Explore content
- About the journal
- Publish with us
- Sign up for alerts
- Review Article
- Published: 04 January 2023
Immune responses to human fungal pathogens and therapeutic prospects
- Michail S. Lionakis ORCID: orcid.org/0000-0003-4994-9500 1 ,
- Rebecca A. Drummond ORCID: orcid.org/0000-0001-5424-7074 2 , 3 &
- Tobias M. Hohl ORCID: orcid.org/0000-0002-9097-5412 4 , 5 , 6 , 7
Nature Reviews Immunology volume 23 , pages 433–452 ( 2023 ) Cite this article
28k Accesses
47 Citations
383 Altmetric
Metrics details
- Antimicrobial responses
- Fungal host response
- Fungal infection
Pathogenic fungi have emerged as significant causes of infectious morbidity and death in patients with acquired immunodeficiency conditions such as HIV/AIDS and following receipt of chemotherapy, immunosuppressive agents or targeted biologics for neoplastic or autoimmune diseases, or transplants for end organ failure. Furthermore, in recent years, the spread of multidrug-resistant Candida auris has caused life-threatening outbreaks in health-care facilities worldwide and raised serious concerns for global public health. Rapid progress in the discovery and functional characterization of inborn errors of immunity that predispose to fungal disease and the development of clinically relevant animal models have enhanced our understanding of fungal recognition and effector pathways and adaptive immune responses. In this Review, we synthesize our current understanding of the cellular and molecular determinants of mammalian antifungal immunity, focusing on observations that show promise for informing risk stratification, prognosis, prophylaxis and therapies to combat life-threatening fungal infections in vulnerable patient populations.
Similar content being viewed by others
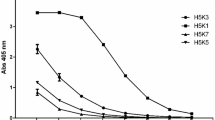
A new humanized antibody is effective against pathogenic fungi in vitro
Tomas Di Mambro, Tania Vanzolini, … Mauro Magnani
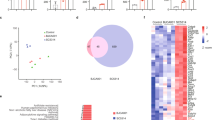
Innate immune responses against the fungal pathogen Candida auris
Yuanyuan Wang, Yun Zou, … Dongsheng Zhou
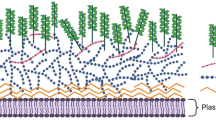
Vaccines for human fungal diseases: close but still a long way to go
Lorena V. N. Oliveira, Ruiying Wang, … Stuart M. Levitz
Introduction
Of the approximately five million fungal species, only a few regularly infect humans. However, in recent decades, the AIDS pandemic and the increasing number of patients who have received myeloablative chemotherapy and/or solid organ or haematopoietic stem cell transplantation (HSCT) have led to a marked increase in the global burden of fungal disease in these immunocompromised individuals (Table 1 and Supplementary Table 1 ). Furthermore, new at-risk populations have emerged, including patients with severe respiratory virus-associated mould disease and individuals being treated with novel, precision immune-targeted biologics (monoclonal antibodies or small-molecule kinase inhibitors) for autoimmune, inflammatory and/or neoplastic disorders 1 , 2 , 3 . Emerging fungi such as multidrug-resistant Candida auris present new threats to public health 4 (Box 1 ). Fungi exhibit distinct morphotypes (such as yeast and hyphae ) and can cause superficial and life-threatening invasive infections, primarily in immunocompetent individuals and immunocompromised individuals, respectively (Table 1 and Supplementary Table 1 ).
Thus, interest in fungal immunology research has increased in recent years, catalysed by our growing understanding of the biology and functions of C-type lectin receptors (CLRs) 5 , 6 , which are the main class of fungal-sensing pattern recognition receptors (reviewed in ref. 5 ). Notably, humans with inherited deficiency of the CLR adaptor protein CARD9 are susceptible to severe mucocutaneous and invasive fungal disease, without developing other infectious or non-infectious complications; this selective susceptibility to fungal infection is a unique feature among the more than 450 known inborn errors of immunity (see later) 7 . In this Review, we synthesize immunological insights from the expanding number of inborn errors of immunity that predispose to fungal infection (Table 2 and Supplementary Table 2 ) and from fungal infection-promoting, immune-targeted biologics (Table 3 and Supplementary Table 3 ), together with mechanistic insights from animal models of fungal disease. We focus on observations that have translational implications for informing risk stratification, prognosis, treatment and vaccination of fungus-infected individuals. The text is structured according to the clinical disease phenotypes that are caused by various pathogenic yeasts, moulds and dimorphic endemic fungi.
Box 1 Candida auris
Since 2009, when it was initially identified in Japan, Candida auris has rapidly spread across six continents, causing multiple outbreaks of skin colonization and subsequent life-threatening bloodstream infections in vulnerable patients; C. auris accounts for up to 30% of cases of candidaemia in certain health care settings 4 , 185 , 186 . C. auris is a major threat to public health owing to its unique propensity for long-term skin colonization, antimicrobial resistance and high person-to-person transmissibility within health care facilities, leading the US Centers for Disease Control and Prevention to designate it as an urgent threat in 2019 (ref. 187 ). The predilection of C. auris to colonize the skin, which distinguishes it from Candida albicans and other Candida species that reside within the gastrointestinal tract, has been verified both ex vivo in human and pig skin models and in vivo in mouse skin, where it establishes long-term residence within deep skin tissue and hair follicles 188 , 189 . C. auris has structurally unique mannoproteins compared with other Candida species, and its immune recognition by human circulating mononuclear cells and their induction of cytokine production is primarily mediated by complement receptor 3 and the mannose receptor, not dectin 1 (ref. 190 ). In a mouse model of skin colonization, IL-17A and IL-17F, but not IL-22, produced by innate and adaptive lymphocytes were crucial for curtailing C. auris skin colonization 189 , and chlorhexidine application ameliorated fungal burden 189 , which is in keeping with a similar finding in human patients 185 . In invasive candidiasis in mice, C. auris is less lethal than Candida albicans 189 , 190 , 191 , yet neutrophils have been shown to be poorly recruited to sites of C. auris infection and to ineffectively produce neutrophil extracellular traps and kill C. auris relative to C. albicans , which suggests that C. auris has specific immune-evading properties that are driven by its unique mannoprotein structure 191 . A better understanding of the fungal and host determinants of the unique skin tropism of C. auris may help to develop improved preventive and therapeutic strategies against this pathogen.
Mucocutaneous candidiasis
Candida species (primarily Candida albicans ) are commensal yeasts of the gastrointestinal and reproductive tracts in most humans. When disruptions to the immune system and/or the resident microbiota occur, Candida can cause opportunistic infections at mucocutaneous or deep-seated anatomical sites, which have different requirements for immune defence 8 (Table 1 ). Specifically, whereas innate and adaptive lymphocytes mediate protection against mucocutaneous candidiasis, myeloid phagocytes are crucial for protection against invasive candidiasis 9 . Indeed, patients with HIV/AIDS, having low CD4 + T cell counts, are at risk of developing mucocutaneous candidiasis, whereas patients with neutropenia are susceptible to invasive candidiasis, but not vice versa 8 .
Mucocutaneous candidiasis presents clinically as oropharyngeal candidiasis (OPC), oesophageal candidiasis or vulvovaginal candidiasis (VVC). A major breakthrough in the field of fungal immunology has been the discovery that IL-17 receptor (IL-17R) signalling is a crucial defence pathway against mucocutaneous candidiasis 10 (Fig. 1a ). Mice and humans with complete genetic deficiencies in IL-17RA, IL-17RC or their adaptor protein ACT1 (also known as TRAF3IP2) are highly susceptible to fully penetrant chronic mucocutaneous candidiasis (CMC) 10 , 11 , 12 , 13 , 14 , 15 , 16 . Several other CMC-manifesting inborn errors of immunity exhibit varying degrees of impaired IL-17 cellular responses and/or decreased frequencies of circulating T helper 17 (T H 17) cells 9 , 17 (Table 2 and Supplementary Table 2 ). Moreover, administration of IL-17 pathway-targeting biologics for the treatment of psoriasis has been associated with mild, treatment-responsive OPC, with a mean frequency of ~2–12%, depending on the biologic agent 18 , 19 (Table 3 ). The relatively low frequency of OPC and the lack of CMC in these patients likely reflect that mucosal IL-17 responses are not completely inhibited by these biologics and are consistent with the notion that profound and sustained inhibition of the IL-17 pathway is required for the development of mucosal fungal disease 18 .
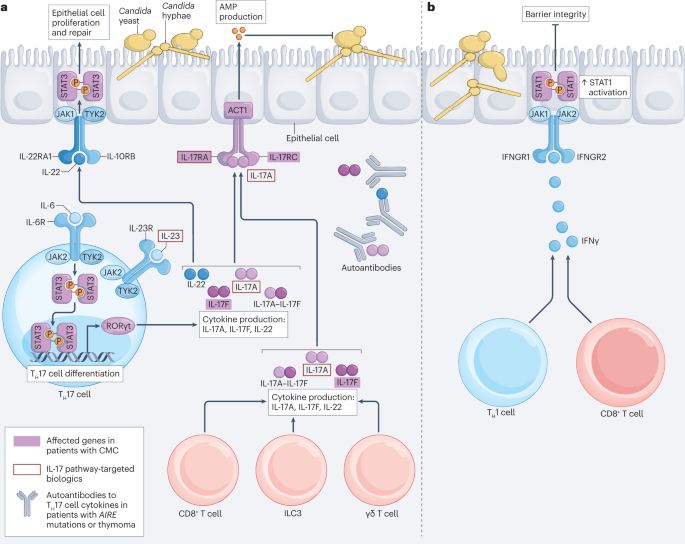
a , Protective responses to Candida at mucosal surfaces are mediated by IL-17 and IL-22 produced by T helper 17 (T H 17) cells, CD8 + T cells, type 3 innate lymphoid cells (ILC3s) and γδ T cells. T H 17 cell differentiation depends on signal transducer and activator of transcription 3 (STAT3)-mediated retinoic acid receptor-related orphan receptor-γt (RORγt) induction downstream of signalling through IL-6 receptor (IL-6R) and IL-23R, which is defective in individuals with mutations in RORC (which encodes RORγt), STAT3 or ZNF341 (which regulates STAT3 expression and function), making them highly susceptible to chronic mucocutaneous candidiasis (CMC). T H 17 cells produce IL-17A, IL-17F and IL-22. IL-17A, IL-17F and IL-17A–IL-17F bind to IL-17R, consisting of IL-17RA and IL-17RC, on suprabasal epithelial cells, which leads to the activation of ACT1 and induces the production of antimicrobial peptides (AMPs; such as β-defensin 3 and S100A8/S100A9) that restrict Candida growth. CMC also develops in patients with mutations in IL17F , IL17RA , IL17RC or TRAF3IP2 (which encodes ACT1), and in patients with thymoma or AIRE mutations, both of which are associated with autoantibodies to IL-17 and/or IL-22. Furthermore, mild oral thrush can develop in a subset of patients receiving IL-17 pathway-targeted biologics such as those inhibiting IL-17A, IL-17RA, IL-12p40 (not shown) or IL-23 (Table 3 ). IL-22 produced by T H 17 cells, CD8 + T cells, ILC3s and γδ T cells binds to the IL-22 receptor consisting of IL-22RA1 and IL-10RB on basal epithelial cells, which activates STAT3 and facilitates epithelial cell proliferation and repair, thereby enabling the replenishment and responsiveness of the IL-17R-expressing suprabasal epithelial cell layer. b , Mucosal type II interferonopathy. In the setting of autoimmunity caused by autoimmune regulator (AIRE) deficiency, mucosal CD4 + T H 1 cells and CD8 + T cells locally produce increased levels of interferon-γ (IFNγ), which binds to the IFNγ receptor consisting of IFNGR1 and IFNGR2 on epithelial cells, activates STAT1 and impairs oral epithelial barrier integrity. This leads to increased susceptibility to oropharyngeal candidiasis. JAK, Janus kinase; TYK2, tyrosine kinase 2.
At the cellular level, IL-17A and IL-17F are produced by αβ T cells, γδ T cells and type 3 innate lymphoid cells during OPC 14 , 15 (Fig. 1a ). The C. albicans toxin candidalysin , Langerin-expressing dendritic cells (DCs) and microbiota-derived retinoic acid prime natural T H 17 cell responses initially, followed by Candida -specific T H 17 cell generation that is facilitated by antigen presentation by CCR2 + monocyte-derived DCs 10 , 20 , 21 , 22 . By contrast, the C. albicans lipase Lip2 was recently shown to suppress IL-17 responses by γδ T cells indirectly through inhibition of IL-23 production by tissue-resident DCs 23 . Notably, mucosal CD8 + T cells, γδ T cells and type 3 innate lymphoid cells can compensate for IL-17 production when T H 17 cells are absent, as Cd4 −/− mice are not susceptible to OPC in primary or recall models and patients with loss-of-expression CD4 mutations or idiopathic CD4 lymphocytopenia who lack CD4 + T cells are resistant to CMC 18 , 24 , 25 , 26 . Thus, decreased levels of circulating T H 17 cells may not in isolation be a reliable immunological biomarker for risk assessment of human CMC, and the direct evaluation of global mucosal IL-17 responses may be needed to determine the mechanisms of CMC.
Following IL-17RA–IL-17RC engagement on epithelial cells by IL-17A and/or IL-17F, the generation of antimicrobial peptides restricts local Candida growth and epithelial invasion 10 , 13 , 20 . Furthermore, the epithelial cell pattern recognition receptor EPHA2 recognizes fungal β-glucan, activates STAT3 and epidermal growth factor receptor 2 (EGFR2) signalling, and boosts mucosal IL-17 responses likely through priming the production of IL-1α/IL-1β during mucosal infection, which facilitates natural T H 17 cell proliferation 20 , 22 , 27 . IL-22, another type 17 cytokine produced by lymphocytes, binds to IL-22RA1–IL-10RB on epithelial cells, activates STAT3 and promotes epithelial cell proliferation and repair, thereby replenishing IL-17R-expressing epithelial cells and enabling their responsiveness to IL-17A and IL-17F 28 (Fig. 1a ).
Whereas IL-17 is protective during OPC, in certain settings — such as the autoimmune syndrome of AIRE deficiency, which features an incompletely penetrant association between CMC and IL-17-neutralizing autoantibodies 18 , 29 , 30 — excessive interferon-γ (IFNγ) production by mucosal αβ T cells can promote OPC susceptibility by driving epithelial barrier disruption, which is ameliorated by IFNγ inhibition or inhibition of JAK–STAT signalling downstream of the IFNγ receptor 31 (Fig. 1b ). This finding suggests that OPC susceptibility could be classified across a spectrum that encompasses impaired type 17 mucosal defences and/or immunopathology-promoting type 1 mucosal inflammation. Indeed, the recent discovery of CMC-manifesting inborn errors of immunity with intact or enhanced IL-17 responses in the setting of autoinflammation or autoimmunity (Supplementary Table 2 ) may uncover additional conditions in which OPC may be driven by mucosal immunopathology rather than by impaired host resistance. Notably, in patients with STAT1 gain-of-function mutations, who universally develop CMC associated with decreased circulating T H 17 cells and increased IFNγ cellular responses, pharmacological JAK–STAT inhibition ameliorates CMC, pointing to a beneficial immunotherapeutic strategy in this setting 32 .
VVC occurs at least once in ~75% of healthy women during their reproductive years. An estimated 6–9% of these women have more than three recurrent episodes of VVC per year, a pervasive condition termed ‘recurrent VVC’. As opposed to OPC, VVC is characterized by maladaptive, fungus-driven, neutrophil-associated inflammation with excessive local production of alarmins and pro-inflammatory cytokines 33 . Correspondingly, genetic polymorphisms in NLRP3, SIGLEC15, IDO1 and other genes that augment the vaginal inflammatory milieu are associated with a greater risk of recurrent VVC 33 , 34 . Interestingly, whereas patients with HIV/AIDS and patients receiving IL-17 pathway-targeted biologics are at increased risk of developing OPC, they are not at risk of developing VVC, in agreement with the finding that IL-17R deficiency does not impair fungal control during mouse VVC 35 . By contrast, antibiotics predispose women to VVC, but not OPC, which indicates that the vaginal microbiota has a role in restricting local growth of Candida through poorly defined mechanisms that require further investigation 36 . Thus, the oral and vaginal mucosal immune responses to C. albicans are distinct. Importantly, VVC has been a target disease for protection with the C. albicans agglutinin-like sequence 3 (Als3)-based vaccine, which was shown to be immunogenic and efficacious in mouse models of VVC and in women with recurrent VVC 37 , 38 . The Als3-based vaccine is the only fungal vaccine that has thus far exhibited clinical protection in humans 37 (Box 2 ).
C. albicans is effectively controlled at the cutaneous barrier by an IL-17-enforced neuroimmune axis and rarely causes clinical skin disease in humans 39 . Specifically, cutaneous C. albicans infection in mice promotes the activation of TRPV1 + sensory neurons expressing the neuropeptide calcitonin gene-related peptide, which is both necessary and sufficient to drive protective IL-17 responses 39 , 40 . These neuroimmune findings paved the way for the recent demonstration that gut fungi drive IL-17-dependent neuroimmune modulation of mouse behaviour 41 , further expanding our knowledge of the essential roles of neuroimmune circuits in tissue homeostasis and immunity (reviewed in ref. 42 ).
Box 2 Fungal vaccines
Despite the substantial global burden, cost, morbidity and mortality associated with opportunistic fungal infections, no human fungal vaccine has been licensed to date. Major challenges in developing human fungal vaccines include concerns about their safety and efficacy in the immunocompromised patients who are typically at risk of serious fungal disease and difficulties in attracting pharmaceutical industry interest. Promising results have been obtained with various vaccine formulations (for example, killed or live attenuated fungi, crude fungal extracts, recombinant fungal subunits and nucleic acids encoding fungal antigens) in animal models of candidiasis, aspergillosis, cryptococcosis, coccidioidomycosis, histoplasmosis and blastomycosis (reviewed elsewhere 192 , 193 ). Among these, only three have reached human clinical trials. The first-in-human fungal vaccine was a formalin-killed spherule vaccine for coccidioidomycosis, which showed no efficacy and caused topical and systemic adverse reactions in a phase III trial in the 1980s 194 . The other two are recombinant Candida vaccines. The first vaccine, termed ‘PEV7’, was based on recombinant C. albicans secreted aspartic protease 2 (Sap2) formulated with influenza virosomes. It protected rats from vulvovaginal candidiasis, and a phase I clinical trial found it to be safe and immunogenic, but no further clinical development has occurred since 2012 (ref. 195 ). The second vaccine, termed ‘NDV-3’, is based on recombinant amino terminus of C. albicans agglutinin-like sequence 3 (Als3) formulated with alum adjuvant. NDV-3 protected mice against mucosal and invasive candidiasis caused by several Candida species, including C. auris , cross-protected against Staphylococcus aureus owing to structural homology between Als3 and an S. aureus clumping factor, and stimulated T helper 1 (T H 1) cell, T H 17 cell and B cell responses 38 , 196 , 197 , 198 . In a phase I clinical trial, NDV-3 was safe and immunogenic, eliciting antigen-specific IgA and IgG and T H 1 cell- and T H 17 cell-associated cytokine responses 199 . In a recent double-blind, placebo-controlled phase Ib/IIa trial involving 188 women with recurrent vulvovaginal candidiasis, the vaccine was safe and immunogenic, and it decreased the frequency of vulvovaginal candidiasis in women older than 40 years 37 . A forthcoming goal is to test the efficacy of the vaccine in preventing candidaemia in high-risk patients in intensive care. Moving forward, harnessing fungal cell wall constituents as adjuvants and exploiting conserved fungal epitopes to induce cross-protection against multiple fungi are exciting strategies towards pan-fungal vaccine development 192 . For example, vaccine delivery of the conserved Blastomyces -derived chaperone calnexin in glucan particles protected mice against endemic dimorphic fungi, aspergillosis, chromoblastomycosis and Pseudogymnoascus destructans , which causes the life-threatening white-nose syndrome in bats 146 , 200 , 201 .
Invasive candidiasis
Invasive candidiasis is a leading cause of nosocomial bloodstream infection in higher-income countries, with mortality that can exceed 40% despite treatment 8 . It typically occurs in patients who are critically ill in whom Candida transitions from a commensal pathogen to an opportunistic pathogen by translocating into the bloodstream and seeding deep-seated organs such as liver, spleen and kidney 8 . Although C. albicans is most often responsible for invasive candidiasis, Candida glabrata , Candida tropicalis and Candida parapsilosis also cause disease with increasing frequency, and the multidrug-resistant Candida auris has spread rapidly worldwide in recent years 8 (Box 1 ).
Certain iatrogenic factors are typically associated with the development of invasive candidiasis in humans 8 . Broad-spectrum antibiotics induce gut dysbiosis, impair lymphocyte-mediated IL-17A-dependent and granulocyte–macrophage colony-stimulating factor (GM-CSF)-dependent intestinal immune responses and inhibit the local generation of antimicrobial peptides, thereby enhancing Candida gut colonization and invasion 43 , 44 ; this antibiotic-induced susceptibility was ameliorated by IL-17A or GM-CSF immunotherapy in a mouse model of invasive candidiasis 44 . Central venous catheters or chemotherapy-induced mucositis and surgery, which breach the integrity of the cutaneous barrier or the intestinal barrier, respectively, can allow Candida to translocate into the bloodstream 8 . Iatrogenic immunosuppression (for example, chemotherapy-induced neutropenia or use of corticosteroids), which impairs protective myeloid phagocyte-dependent antifungal responses, facilitates the invasion of deep-seated organs by Candida 8 . Rapid neutrophil influx and activation in Candida -infected tissues are crucial for effective host defence in mice, in agreement with the profound susceptibility of humans with neutropenia to invasive candidiasis 8 .
The CLRs dectin 1 (also known as CLEC7A), dectin 2 (also known as CLEC6A) and dectin 3 (also known as CLEC4D) recognize Candida β-glucans and α-mannans and signal through the kinase SYK and the CARD9–MALT1–BCL-10 signalling complex to activate NF-κB, collectively promoting T H 17 cell development and pro-inflammatory cytokine production 5 , 8 . CARD9 deficiency is so far the only known inborn error of immunity that predisposes to both mucosal candidiasis and invasive candidiasis, with a unique tropism for the central nervous system (CNS) 45 (Table 2 ). Mechanistically, in response to fungal candidalysin, CNS-resident microglia produce IL-1β in a CARD9-dependent manner, which mediates protective neutrophil recruitment to the CNS through CXCL1–CXCR2-dependent chemotaxis 46 . In line with this, pharmacological blockade of the CLR–SYK–CARD9 signalling axis with the SYK inhibitor fostamatinib has been associated with opportunistic fungal infections (Supplementary Table 3 ).
Invasive candidiasis also occurs in a small proportion of patients with severe congenital neutropenia syndromes or inborn errors of immunity that affect the phagocyte oxidative burst, such as complete myeloperoxidase deficiency or chronic granulomatous disease (caused by mutations in NADPH oxidase complex subunits) 9 (Table 2 and Supplementary Table 2 ). Although invasive candidiasis is not a recognized phenotype of inherited deficiency of complement component C5 (ref. 9 ), acute pharmacological inhibition of C5a with eculizumab increases susceptibility to Candida infection, which suggests a crucial role of complement activation in mounting innate antifungal responses in vulnerable patients 47 (Supplementary Table 3 ).
Within Candida -infected tissues, an intricate intercellular crosstalk orchestrates the candidacidal activity of neutrophils. Specifically, Ly6C + inflammatory monocytes and monocyte-derived DCs produce IL-15 and IL-23, respectively, which in turn activate natural killer cells to release GM-CSF, which primes neutrophils to kill Candida 48 , 49 . Consistent with these results, a phase IV clinical trial showed that GM-CSF administration in allogeneic HSCT recipients decreased the incidence of invasive candidiasis and associated mortality 50 . Neutrophil reactive oxygen species (ROS)-dependent killing of Candida involves dectin 1-dependent metabolic rewiring of neutrophils and dectin 1-mediated activation of the calcineurin–NFAT and MAC1 (CD11B–CD18)–VAV–PKCδ pathways 8 , 51 . Uraemia , which is another known risk factor for human invasive candidiasis, impairs neutrophil ROS production and Candida killing by reducing GLUT1-dependent glucose uptake through hyperactivation of glycogen synthase kinase-3β (GSK3β) 52 . Pharmacological inhibition of GSK3β restores neutrophil candidacidal activity in mice and humans with uraemia, pointing to a potential therapeutic intervention in this setting 52 .
Although neutrophils are protective during invasive candidiasis, their excessive accumulation and activation may also have detrimental effects, as seen in patients with neutropenia upon neutrophil recovery. Corticosteroids ameliorate inflammation and clinical symptoms in this setting, but certain immunopathogenic factors have been delineated in mice and humans — for example, the chemokine receptor CCR1, the CLR DNGR1, the endoribonuclease MCPIP1, the secreted growth factor progranulin and IFNγ — that show promise for eventually developing more selective therapeutic approaches 9 , 53 , 54 , 55 , 56 , 57 . Moreover, immunopathology during renal candidiasis can be ameliorated through activation of the IL-17-dependent kallikrein–kinin system , which prevents renal tubular cell apoptosis and preserves kidney function 58 . Administration of bradykinin combined with antifungal therapy provided a synergistic survival benefit during mouse kidney infection with Candida 58 , raising the possibility that the antihypertensive class of angiotensin-converting enzyme inhibitors, which increase bradykinin levels, might have protective effects in this context.
In mouse invasive candidiasis, in addition to neutrophils, CCR2 + infiltrating monocytes and CX3CR1 + tissue-resident macrophages also contribute to protective immunity in the kidney and CNS by promoting Candida uptake and killing 59 , 60 . Importantly, beyond acute experimental candidiasis, monocytes and macrophages also protect against systemic C. albicans reinfection through a process termed ‘ trained immunity ’. Mechanistically, protection in this setting is conferred through activation of dectin 1, RAF1, AKT, mTOR and hypoxia-inducible factor 1α (HIF1α) signalling that drives epigenetic reprogramming and cellular metabolic rewiring of monocytes and macrophages, with a switch to aerobic glycolysis 9 , 61 . By contrast, activation of macrophage JNK1 increases mortality during invasive candidiasis by downregulating expression of the CLR CD23 (also known as FcεRII) and impairing macrophage production of nitric oxide 62 . Thus, whereas JNK1 deficiency predisposes to CMC in humans (Supplementary Table 2 ), it may protect against invasive candidiasis 62 , 63 . Interestingly, although patients with humoral defects are not considered at risk of bloodstream candidiasis, mouse gut-educated IgA + meningeal plasma cells located near dural venous sinuses were recently shown to entrap C. albicans and restrict its spread in the CNS, uncovering a potential role for humoral immunity in protection against invasive candidiasis at this anatomical site 64 .
Population studies in patients who are critically ill have shown that genetic polymorphisms in genes including TLR1 , CXCR1 , CX3CR1 , STAT1 and TAGAP , many of which have shown corroborating protective effects in mouse models of invasive candidiasis, are associated with greater risk of invasive candidiasis and/or worse outcome after infection. Notably, the combined presence of certain polymorphisms can increase susceptibility to invasive candidiasis by up to ~20-fold 8 , 65 . Collectively, these findings may provide a foundation for the eventual development of immunogenomic approaches for personalized risk stratification, prophylaxis and prognostication in high-risk patients.
Aspergillosis
Aspergillosis is an opportunistic, life-threatening fungal infection that develops following inhalation of ubiquitous Aspergillus moulds (primarily Aspergillus fumigatus ) in humans with numeric or functional deficits in myeloid phagocytes, such as individuals with neutropenia, who receive corticosteroids or who are HSCT recipients, and, recently, in patients with severe influenza or COVID-19 pneumonia 1 , 3 , 66 (Table 1 ). Humans continuously inhale Aspergillus conidia (2–3 μm in diameter in the case of A. fumigatus ), and an important immunological checkpoint involves preventing the formation of tissue-invasive filamentous hyphae in the respiratory tree. Myeloid phagocytes are necessary and sufficient to maintain this checkpoint as Rag2 −/− Il2rg −/− mice that lack innate and adaptive lymphocytes clear conidia without developing invasive disease 67 , similarly to humans with defects in lymphoid function or numbers 9 . Although lymphocytes seem to be redundant for protection against aspergillosis in hosts with intact myeloid cells, it is possible that adoptively transferred lymphocytes may provide benefit in hosts with an injured myeloid compartment 68 , 69 . Aspergillus also causes allergic disease and chronic cavitation in individuals with atopy and structural lung damage, respectively (reviewed in refs. 70 , 71 ).
A central theme of defence against inhaled Aspergillus is molecular and cellular redundancy in achieving sterilizing immunity and an essential role of intercellular crosstalk to regulate leukocyte infiltration and effector functions within the lung (Fig. 2 ). In immunocompetent individuals, conidia are engulfed by alveolar macrophages 72 and conidial germination within phagosomes triggers a pro-inflammatory cascade through dectin 1-dependent recognition of β-1,3-glucan 73 , which is exposed on the surface of the germinating conidia 74 . Moreover, exposure of fungal mannans engages soluble pentraxin 2 (ref. 75 ) and pentraxin 3 (ref. 76 ) and the CLRs dectin 2 and dectin 3, sequentially activating SYK, CARD9–MALT1–BCL-10, NF-κB and the NLRP3 inflammasome, and thereby resulting in pro-inflammatory cytokine production 9 . CLR-dependent activation of phospholipase-γ induces Ca 2+ –calcineurin phosphatase activity and nuclear translocation of the transcription factor NFAT 9 . In addition to these immunostimulatory Aspergillus polysaccharides, conidial dihydroxynaphthalene melanin activates the CLR MelLec (also known as CLEC1A) and induces downstream signalling through an unknown pathway 77 . The Aspergillus thaumatin-like protein CalA binds to epithelial and endothelial cells through α5β1 integrin to promote its internalization and spread, and antibody-mediated blockade of CalA ameliorates mortality in Aspergillus -infected mice 78 .
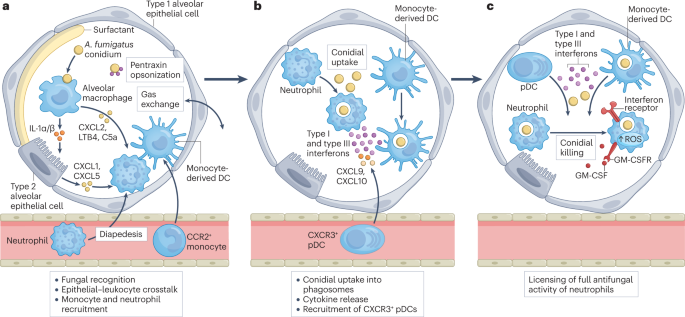
a , Fungal recognition and epithelial cell–leukocyte crosstalk coordinate the recruitment of neutrophils and monocytes to the alveoli. Alveolar macrophages and lung-resident dendritic cells (DCs; not shown) engulf Aspergillus fumigatus conidia that reach the terminal airways, which are the site of gas exchange. Rapid release of IL-1α/β by macrophages and DCs stimulates the IL-1 receptor (IL-1R)–MYD88-dependent production of the neutrophil-recruiting chemokines CXCL1 and CXCL5 by lung epithelial cells. The release of CXCL2 by haematopoietic cells, including macrophages, through C-type lectin receptor (CLR)–CARD9 activation, and the rapid production of leukotriene B 4 (LTB4) and complement component C5a also contribute to sustained neutrophil recruitment. In addition, CCR2 + circulating monocytes enter the lung parenchyma and differentiate into monocyte-derived DCs. Opsonization of A. fumigatus conidia by pentraxins enables their efficient uptake and killing by neutrophils. b , The recruited neutrophils and monocyte-derived DCs engulf conidia into phagolysosomes, which are the site of intracellular conidial killing. Both resident myeloid cells and infiltrating CCR2 + monocytes produce type I interferon early in the response and regulate ensuing type III interferon release. Fungus-engaged neutrophils and monocyte-derived DCs secrete CXCL9 and CXCL10 (through CLR–CARD9–SYK and type I interferon-dependent pathways, respectively), which recruit CXCR3 + plasmacytoid DCs (pDCs) to the lung. c , Innate crosstalk licenses the full spectrum of neutrophil antifungal activity. Conidial killing by neutrophils and monocyte-derived DCs is enhanced by the entry of CXCR3 + pDCs and by type I and type III interferons that converge on signal transducer and activator of transcription 1 (STAT1) signalling in neutrophils. Fungus-induced release of granulocyte–macrophage colony-stimulating factor (GM-CSF) also potentiates neutrophil antifungal properties by enhancing NADPH oxidase activity and the production of reactive oxygen species (ROS). In the context of blastomycosis, CCR6 + innate lymphocytes and natural T helper 17 cells contribute to GM-CSF production during acute pulmonary infection.
Among the inborn errors of immunity, aspergillosis is a signature infection of chronic granulomatous disease 9 , which highlights the importance of ROS generation in maintaining sterilizing lung immunity (Table 2 ). Aspergillus hyphae induce neutrophil production of ROS through β2 integrin and SYK 79 , which triggers a regulated cell death process in Aspergillus that is opposed by the fungal anti-cell-death gene Afbir1 (ref. 80 ). On the basis of the fact that IFNγ stimulates phagocyte ROS production, recombinant IFNγ has been approved for use as an immunotherapy to prevent invasive (including fungal) infections in patients with chronic granulomatous disease 81 . In addition to licensing neutrophil fungicidal activity, ROS also regulate LC3-associated phagocytosis , an adjunctive mechanism of fungal clearance 82 . Aspergillus conidial melanin hinders dectin 1-dependent and SYK-dependent activation of LC3-associated phagocytosis in macrophages, and phagosomal removal of melanin enhances macrophage antifungal activity by increasing calcium flux through glycolysis 83 .
In pulmonary aspergillosis in mice, infiltrating neutrophils, Ly6C + inflammatory monocytes and CXCR3 + plasmacytoid DCs are essential cellular effectors 67 , 84 (Fig. 2 ). Rapid neutrophil influx depends on sequential IL-1R–MYD88 signalling-dependent release of CXCL1 and CXCL5 by the lung epithelium, including club cells 85 , 86 . Thereafter, CLR–CARD9-dependent signalling in haematopoietic cells, including macrophages, sustains pulmonary neutrophil influx through CXCL2 production, which is consistent with a model of sequential and compartment-specific regulation of pulmonary neutrophil recruitment in this context. In CARD9-deficient humans, aspergillosis is typically extrapulmonary, which supports the idea that CARD9-dependent and CARD9-independent pathways of neutrophil recruitment collaborate in the lung, but that CARD9 predominantly controls neutrophil recruitment to extrapulmonary sites of Aspergillus infection 87 . In addition to IL-1R–MYD88-dependent and CLR–CARD9-dependent leukocyte recruitment to the Aspergillus -infected lung, signalling through the RNA sensor MDA5–MAVS 88 and leukotriene B 4 biosynthesis 89 also contribute. Within the Aspergillus -infected lung, the lectin family member galectin 3 increases neutrophil motility and facilitates neutrophil extravasation into bronchoalveolar air spaces 90 .
In addition to neutrophils, mononuclear phagocytes also promote pulmonary defences against Aspergillus both directly and indirectly by priming neutrophil effector functions. CCR2 + lung-infiltrating monocytes differentiate into monocyte-derived DCs that engulf, inactivate and transport conidia to lung-draining lymph nodes 67 , 91 . CCR2 + monocyte-derived DCs also produce type I interferons, which in turn control type III interferon production within the fungus-infected lung. Type I and type III interferon receptor engagement and STAT1 signalling are essential for host defence by enhancing neutrophil ROS production and fungicidal activity, and JAK–STAT inhibitors have been reported to promote aspergillosis in humans 92 (Table 3 ). In addition, STAT1 signalling in CCR2 + monocytes facilitates the formation of monocyte-derived DCs and enhances their fungicidal activity; this process is supported by reciprocal interferon–STAT1 crosstalk with neutrophils 93 . Fungus-induced release of GM-CSF also stimulates neutrophil ROS production and promotes fungal clearance 94 . Concordantly, humans with mutations in GM-CSF receptor signalling develop pulmonary alveolar proteinosis, a condition that is associated with opportunistic pulmonary infections, including aspergillosis 95 (Supplementary Table 2 ).
Upon conidial engulfment, lung-infiltrating neutrophils and monocytes produce CXCR3-targeted chemokines (CXCL9 and CXCL10) to recruit circulating CXCR3 + plasmacytoid DCs into the lung, where they boost neutrophil ROS production, neutrophil extracellular trap formation and fungicidal activity 84 . Thus, both epithelial cell-derived chemokines and haematopoietic cell-derived chemokines coordinate early neutrophil influx to the Aspergillus -infected lung, and tissue-infiltrating mononuclear phagocytes license neutrophil functions to achieve sterilizing immunity. Nutrient limitation via metal chelators (for example, lactoferrin or calprotectin) also contributes to tissue-specific control of Aspergillus , with a role shown for calprotectin in protecting against Aspergillus keratitis but not pulmonary infection in mice 96 , 97 . Corroborating these mechanistic studies of protective immunity in mice, human population studies have identified genetic polymorphisms in APCS (encoding pentraxin 2), PTX3 , CLEC7A (encoding dectin 1), CLEC1A and CXCL10 that predispose to aspergillosis following allogeneic HSCT 75 , 77 , 98 , 99 .
Moreover, pharmacological susceptibility to aspergillosis has provided additional insights into host defence pathways (Table 3 and Supplementary Table 3 ). Chief among these is the observation that ibrutinib-induced inhibition of Bruton’s tyrosine kinase (BTK) promotes aspergillosis in patients with lymphoma 100 , 101 , which is recapitulated in Btk −/− mice, consistent with BTK having an evolutionarily conserved role in defence against Aspergillus 100 . In macrophages, phagocytosis of Aspergillus conidia triggers dectin 1-dependent and Toll-like receptor 9 (TLR9)-dependent activation of BTK, which regulates calcineurin–NFAT activity and synergizes with NF-κB to promote the production of tumour necrosis factor (TNF) 102 . The mechanisms by which BTK mediates neutrophil anti- Aspergillus defence remain poorly defined and require further study.
Mucormycosis
Mucormycosis is a life-threatening infection caused by ubiquitous inhaled moulds of the order Mucorales (primarily Rhizopus species) 103 . As is the case for aspergillosis, mucormycosis typically occurs in patients with quantitative and/or qualitative phagocyte defects, such as individuals with neutropenia, who receive corticosteroids or who are HSCT recipients 103 . However, Mucorales members are unique among the human mould pathogens in terms of their predilection to cause disease in the settings of diabetic ketoacidosis or iron overload 103 (Supplementary Table 1 ). Moreover, mucormycosis has emerged as a devastating complication of COVID-19, particularly in India (where it had a prevalence of 0.27% among hospitalized patients with COVID-19), associated with corticosteroid use and uncontrolled hyperglycaemia 1 . Strikingly, mucormycosis has distinct infection patterns in patients with different risk factors. Specifically, diabetic ketoacidosis typically predisposes to the rhino-orbital–cerebral form of the disease, whereas patients with neutropenia or who are HSCT recipients typically develop sinopulmonary mucormycosis 103 .
During infection in mice, Mucorales members produce mucoricin, a plant ricin-like toxin that promotes cellular necrosis, vascular permeability and death 104 . Recent work has uncovered tissue-specific mechanisms by which hyperglycaemia and iron overload increase susceptibility to mucormycosis. Specifically, increased levels of glucose, iron and ketone bodies, all of which occur during diabetic ketoacidosis, attenuate neutrophil-mediated damage to Rhizopus and induce expression of the glucose-regulated receptor GRP78 on the surface of endothelial cells and nasal epithelial cells and of the Mucorales invasin CotH3 (refs. 105 , 106 , 107 , 108 ). The CotH3–GRP78 interaction enables fungal invasion and damage of the nasal epithelium, and can be targeted with therapeutic benefit in Rhizopus -infected mice with diabetic ketoacidosis 105 , 106 , 107 . Because Aspergillus does not encode CotH3, this functional attribute of Mucorales may underlie the selective predisposition of patients with diabetes to mucormycosis. By contrast, in the setting of pulmonary mucormycosis, Rhizopus CotH7 interacts with β1 integrin, not GRP78, on the surface of alveolar epithelial cells to promote fungal invasion, EGFR activation and cellular damage 108 , 109 . Pharmacological inhibition of β1 integrin or EGFR with the FDA-approved inhibitors cetuximab or gefitinib blocks fungal invasion of alveolar epithelial cells and prolongs mouse survival during pulmonary mucormycosis 108 , 109 . Thus, Rhizopus interacts with different tissue-specific mammalian receptors depending on the underlying predisposing factor to instigate opportunistic disease. Furthermore, during pulmonary mucormycosis in mice, alveolar macrophages contribute to fungal clearance and host survival by stimulating an intracellular iron restriction programme that curbs Rhizopus growth within phagosomes, underscoring the importance of protective nutritional immunity in this setting 110 .
Chromoblastomycosis and phaeohyphomycosis
Chromoblastomycosis is a disfiguring, tumour-like, progressive and often treatment-unresponsive infection in tropical areas, which has been designated as a neglected tropical disease by the World Health Organization. It is primarily caused by the pigmented fungus Fonsecaea pedrosoi , which typically enters the skin via traumatic inoculation (Supplementary Table 1 ). In F. pedrosoi -infected mice, CD4 + T cells are essential for fungal clearance, primarily involving T H 1 cell and T H 17 cell responses 111 , 112 . Unlike Candida and Aspergillus species, F. pedrosoi stimulates modest CLR responses through the dectin 2-dependent and Mincle-dependent SYK–CARD9 axis and fails to effectively activate TLRs, resulting in inadequate local inflammatory responses in infected mice and humans 111 , 113 . Topical administration of the TLR7 agonist imiquimod combined with antifungal therapy markedly improved clinical outcomes in patients with refractory chromoblastomycosis 114 , demonstrating a key adjunctive role for this immunotherapeutic intervention.
Phaeohyphomycosis is another mycosis caused by melanin-bearing, pigmented fungi that typically enter the skin via traumatic inoculation. Although infection is self-limited in most healthy individuals, it can cause severe subcutaneous or disseminated disease in patients who are HSCT recipients or who have CARD9 deficiency 7 , 115 (Supplementary Table 1 ). Exserohilum rostratum , one of the agents of phaeohyphomycosis, caused a meningitis outbreak following epidural injections of contaminated methylprednisolone in the USA in 2012 (ref. 116 ). CARD9-dependent defence against phaeohyphomycosis in mice was recently shown to rely on macrophages, whereas neutrophil recruitment to the infected skin was CARD9 independent 117 . Specifically, CARD9 primes macrophages to produce IL-1β and TNF, which promote macrophage killing of fungi in an interdependent manner 117 . Consistent with this finding, phaeohyphomycosis has been reported following treatment with TNF-targeted biologics such as infliximab 118 (Table 3 ). Upstream of CARD9, dectin 1 is also crucial for macrophage-mediated defence against phaeohyphomycosis in mice, and deleterious mutations in the gene encoding dectin 1 ( CLEC7A ) that impair fungal sensing and TNF production were found in ~70% of putatively immunocompetent patients with severe forms of phaeohyphomycosis 117 . These findings suggest that human dectin 1 deficiency may contribute to susceptibility to severe phaeohyphomycosis in the setting of traumatic inoculation.
Cryptococcosis
Cryptococcosis is caused by the encapsulated yeasts Cryptococcus neoformans and Cryptococcus gattii , which have distinct infection patterns in humans 119 . C. neoformans is more common, and typically causes meningoencephalitis in immunocompromised patients with CD4 + T cell depletion and/or dysfunction (Table 1 ). Cryptococcal meningoencephalitis is an AIDS-defining illness and remains a significant cause of death in resource-limited areas (for example, some parts of Africa) that are associated with limited availability of antiretroviral therapy and first-line antifungal drugs. The incidence of cryptococcal meningoencephalitis is increasing in non-HIV-infected individuals in the Western hemisphere. By contrast, C. gattii primarily causes pulmonary disease in putatively immunocompetent individuals and rarely affects individuals with HIV/AIDS.
Human exposure to inhaled Cryptococcus is widespread by early childhood. The crucial immunological checkpoint for preventing local proliferation of yeast in the lung and extrapulmonary dissemination is the containment and killing of inhaled Cryptococcus by lung-resident macrophages, which is enabled by their crosstalk with IFNγ-producing CD4 + T cells 119 (Fig. 3 ). Notably, Cryptococcus yeasts secrete a capsule containing glucuronoxylomannan and galactoxylomannan that shields its pathogen-associated molecular patterns from immune recognition 119 , 120 . Unsurprisingly, therefore, defective TLR-mediated and CLR-mediated responses in the setting of human MYD88 deficiency and CARD9 deficiency, respectively, do not predispose to cryptococcosis 9 . By contrast, a few inborn errors of immunity primarily affecting numbers of lymphocytes and/or monocytes and macrophages, and/or their activation, underlie human cryptococcosis (Table 2 and Supplementary Table 2 ), which supports the crucial role of effective crosstalk between lymphocytes and mononuclear phagocytes in cryptococcal clearance 9 , 119 .
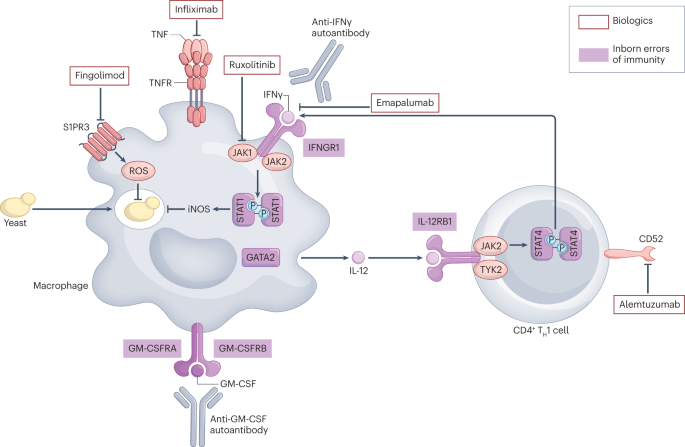
Type 1 immune responses, characterized by interferon-γ (IFNγ)-producing T cells and macrophages, are crucial for protection against intramacrophagic fungi (such as Cryptococcus and Histoplasma ). Infected macrophages produce IL-12, which drives T helper 1 (T H 1) cell polarization of CD4 + T cells through Janus kinase 2 (JAK2)–signal transducer and activator of transcription 4 (STAT4) signalling. T H 1 cells produce IFNγ, which binds to the IFNγ receptor, comprising IFNGR1 and IFNGR2, on macrophages and activates JAK1/JAK2–STAT1-dependent signalling to induce expression of inducible nitric oxide synthase (iNOS). In turn, iNOS mediates fungal killing together with the production of reactive oxygen species (ROS), which is partly controlled by sphingosine 1-phosphate receptor 3 (S1PR3) in the setting of cryptococcal infection. Tumour necrosis factor (TNF) activates macrophages for fungal killing and promotes the formation of granulomas and IFNγ production by lymphocytes. Granulocyte–macrophage colony-stimulating factor (GM-CSF) also primes macrophages to effectively kill fungi intracellularly. The transcription factor GATA2 regulates fungal uptake by macrophages 184 . Disruption of these protective antifungal immune pathways by biologics, inborn errors of immunity or anti-cytokine autoantibodies enhances susceptibility to infections with intracellular fungi in humans.
Loss-of-function mutations in IL12RB1 are associated with cryptococcosis, which is explained by impaired IL-12-driven IFNγ production by T cells and the resulting inability of macrophages to promote intracellular killing of fungi 9 (Fig. 3 ). Consistent with this notion, cryptococcosis develops in adult patients with neutralizing autoantibodies to IFNγ 121 , which may be amenable to anti-CD20 or anti-CD38 targeted biologics that deplete B cells and plasma cells, respectively 122 , 123 . The higher prevalence of autoantibodies to IFNγ in individuals of Asian ancestry who were born in Asia but not elsewhere suggests that both environmental and genetic factors are involved 121 . Moreover, impaired T cell responses promote cryptococcosis in the setting of CD40L deficiency 124 . GATA2 deficiency predisposes to infection with various intramacrophagic pathogens, including mycobacteria and Cryptococcus , associated with profound monocytopenia 125 . GM-CSF is a key factor that promotes anticryptococcal immunity by activating macrophages to a functional state that is associated with efficient fungal killing 126 . Inherited deficiency of GM-CSF receptor signalling or neutralizing autoantibodies to GM-CSF can underlie cryptococcosis, with an enrichment for C. gattii infections in particular 121 , 127 . A genome-wide association study in individuals with HIV/AIDS of African descent uncovered a correlation between CSF1 genetic variation and cryptococcosis 128 . CSF1 encodes macrophage colony-stimulating factor (M-CSF), another growth factor that is crucial for macrophage activation and survival, particularly for CNS-resident microglia 129 .
Pharmacological susceptibility to cryptococcosis has provided additional evidence for the importance of crosstalk between T H 1 cells and macrophages (Table 3 and Supplementary Table 3 ). For example, JAK–STAT inhibitors (which block IFNγ receptor signalling), TNF inhibitors (which impair IFNγ production and granuloma formation) and BTK inhibitors (which impair macrophage activation) all increase the risk of cryptococcosis 9 . Alemtuzumab (which targets CD52 on lymphocytes) and natalizumab (which targets α4β1 integrin on lymphocytes) promote cryptococcosis through profound and prolonged T cell depletion and through impaired integrin-mediated T cell trafficking to Cryptococcus -infected tissues, respectively 9 . The sphingosine 1-phosphate receptor (S1PR) inhibitor fingolimod was recently reported to promote cryptococcosis. In a mouse model of cryptococcosis, fingolimod enhanced susceptibility by impairing S1PR3-dependent macrophage phagocytosis and granuloma formation and by driving a macrophage phenotype with poor oxidative killing capacity, not by affecting S1PR1-mediated T cell trafficking 130 .
Defective IFNγ signalling in macrophages impairs the activity of inducible nitric oxide synthase (iNOS) and hence macrophage oxidative killing pathways, which enables Cryptococcus to survive within phagosomes, where it can be shielded from immune recognition, as has been shown in infected brain tissue of patients with HIV/AIDS 131 . Intracellular growth in macrophages is promoted by fungal-derived urease and modification of the phagosome microenvironment 132 , 133 . In patients with HIV/AIDS, a lack of CD4 + T cells and paucity of pro-inflammatory cytokines (IFNγ and TNF) in the cerebrospinal fluid were the strongest predictors of cryptococcosis severity in the CNS, and T cell responses producing IFNγ and TNF were associated with increased survival 134 , 135 , 136 . Consistent with the idea that IFNγ may boost macrophage antifungal activity in patients with HIV/AIDS in the absence of T cell help, a clinical trial showed that adjunctive IFNγ immunotherapy rapidly reduced fungal burden in the cerebrospinal fluid, the level of which is a known surrogate of patient survival 137 .
Although they are crucial for fungal control, T H 1 cells may also promote pathogenic effects in the settings of non-HIV cryptococcosis and HIV-associated immune reconstitution inflammatory syndrome (IRIS), a condition characterized by the development of life-threatening immunopathology upon initiation of antiretroviral therapy. In these patients, corticosteroids ameliorate inflammatory sequelae and neurological symptoms 138 . In patients without HIV infection with cryptococcosis, significant infiltration of CXCR3 + T H 1 cells into the CNS is observed, associated with high levels of CXCL10 in cerebrospinal fluid, poor colocalization of macrophages and fungus, and neuronal damage 139 . Correspondingly, in a mouse model of non-HIV cryptococcosis, CXCR3 inhibition abrogated the recruitment of T H 1 cells into the CNS by glial-derived CXCL10 and increased survival 140 . Furthermore, patients with AIDS without IRIS have increased numbers of T H 1 cells and increased serum levels of IFNγ relative to patients without IRIS 141 . In a mouse model of cryptococcus-associated IRIS, IFNγ-producing CD4 + T cells were sufficient to mediate CNS damage associated with upregulation of aquaporin 4, which modulates water influx and swelling within the brain 142 . Better understanding of the molecular underpinnings of pathogenic inflammation during cryptococcus-associated IRIS may help to identify targeted, corticosteroid-sparing immunomodulatory strategies for affected patients.
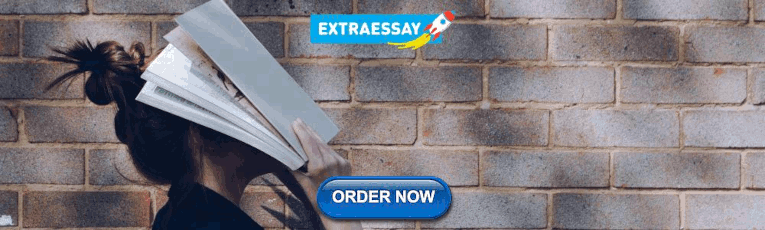
Other endemic fungal infections
Histoplasma , Coccidioides , Paracoccidioides, Talaromyces and Blastomyces species are geographically restricted dimorphic fungi that typically cause self-limited pneumonia in healthy individuals but that can lead to progressive pulmonary and/or extrapulmonary disease in immunocompromised patients with HIV/AIDS, corticosteroid use or transplantation (Table 1 and Supplementary Table 1 ). Race, ethnicity and hormonal factors can influence the risk and severity of these infections, through mechanisms that remain poorly understood. For example, African American, Native American and Asian ancestries are associated with increased risk of blastomycosis and disseminated coccidioidomycosis, pregnancy increases the risk of severe coccidioidomycosis, and chronic paracoccidioidomycosis more commonly affects males 9 .
As for cryptococcosis, defence against histoplasmosis, coccidioidomycosis, paracoccidioidomycosis, talaromycosis and blastomycosis depends on the coordinated crosstalk between IFNγ-producing T cells and fungicidal macrophages (Fig. 3 ). In line with this crucial role of granulomatous inflammation, inborn errors of immunity affecting type 1 immune responses caused by mutations in the genes encoding IL-12RB1, IFNγR1, STAT1, STAT3, CD40L or GATA2 (Table 2 and Supplementary Table 2 ) predispose to severe, disseminated infections by these fungi, as do neutralizing autoantibodies to IFNγ 121 and receipt of TNF inhibitors, JAK–STAT inhibitors or the IFNγ-targeted biologic emapalumab 9 (Table 3 and Supplementary Table 3 ). In Coccidioides -infected patients, genetic variants in β-glucan sensing and response genes (for example, CLEC7A and PLCG2 ) that impair TNF production were recently shown to underlie disseminated disease in ~50% of evaluated patients 143 . By contrast, type 2 immune responses are detrimental for pathogen control by dampening type 1 responses and skewing macrophages towards a phenotype that is permissive for intracellular fungal persistence 144 . Macrophage-mediated nutritional immunity to Histoplasma is also mediated by T H 1 cell responses, particularly GM-CSF, which promotes metallothionein-mediated zinc sequestration that starves the pathogen. By contrast, the T H 2 cell cytokine IL-4 promotes zinc acquisition by yeast, which enables its intracellular survival 145 . The Blastomyces adhesin BAD1 inhibits CD4 + T cell-derived IFNγ release, and Blastomyces uses additional immune-evasion strategies, including cleavage and inactivation of GM-CSF and of monocyte-recruiting CC chemokines 146 .
Immunomodulatory restoration of the T H 1 cell–T H 2 cell balance during endemic mycoses can be exploited with therapeutic benefit. Specifically, a child with no known inborn error of immunity and refractory disseminated coccidioidomycosis who had increased levels of IL-4 and decreased levels of IFNγ achieved rapid symptom resolution and infection remission after adjunctive administration of recombinant IFNγ and the IL-4/IL-13 receptor inhibitor dupilumab 147 .
Pneumocystis jirovecii pneumonia
Pneumocystis jirovecii pneumonia (PJP) is an AIDS-defining illness that develops with declining CD4 + T cell counts and carries a high global disease burden and mortality. In HIV-negative individuals, PJP is most often seen following corticosteroid administration (Table 1 ). Epidemiological data indicate that inhalational exposure to P. jirovecii is universal during infancy without causing severe clinical disease, which is indicative of potent, protective host responses to Pneumocystis 148 . In mice infected with Pneumocystis murina , the mouse-specific Pneumocystis species, CD4 + T cells are crucial for defence as CD4-deficient animals are highly susceptible, which is consistent with the increased risk of PJP in individuals with HIV/AIDS or patients treated with the T cell-depleting biologic alemtuzumab (Table 3 ). Although T H 1 cells, T H 2 cells and T H 17 cells are all induced during mouse infection, none of them has been shown individually to promote disease resolution using corresponding gene-deficient mouse strains 149 . Recently, CD4 + T cell-intrinsic signalling through IL-21R and STAT3 was found to be crucial for defence in Pneumocystis -infected mice, by priming T cell effector functions and protective class-switched antibody responses 150 . These findings recapitulate the susceptibility to PJP that is seen in patients with STAT3 and IL21R mutations (Table 2 and Supplementary Table 2 ). In addition to T cells, B cells are also crucial for anti- Pneumocystis defence through antigen presentation to and priming of CD4 + T cell responses in the lung, by facilitating opsonic phagocytosis by alveolar macrophages, and through secreted IgM 151 , 152 , 153 . In agreement with this role for B cells, recipients of CD20-targeted B cell-depleting antibodies are at risk of developing PJP (Supplementary Table 3 ). Among myeloid phagocytes, alveolar macrophages and eosinophils, but not neutrophils, contribute to Pneumocystis clearance during mouse infection 154 , 155 . Other PJP-manifesting inborn errors of immunity that involve impaired T cell and/or B cell responses include severe combined immunodeficiency, anhidrotic ectodermal dysplasia with immune deficiency due to IKBA deficiency, and mutations in CD40L , IKZF1 or IKZF3 (ref. 149 ) (Supplementary Table 2 ). Biologics that predispose humans to PJP include TNF, PI3K and JAK–STAT inhibitors, which impair the responses of T cells and macrophages to Pneumocystis 149 (Table 3 ).
Endogenous fungi: protection and pathology
Fungi are core constituents of endogenous microbial communities at several human barrier sites (Box 3 ). Recent studies have advanced the concept that tissue-resident fungi shape immune homeostasis and development, function as reservoirs for invasive fungal infections and contribute to the pathogenesis of autoimmune, inflammatory and neoplastic diseases (Fig. 4 ). Here we focus primarily on human studies of the mycobiota, with mouse work having been reviewed elsewhere 156 , 157 .
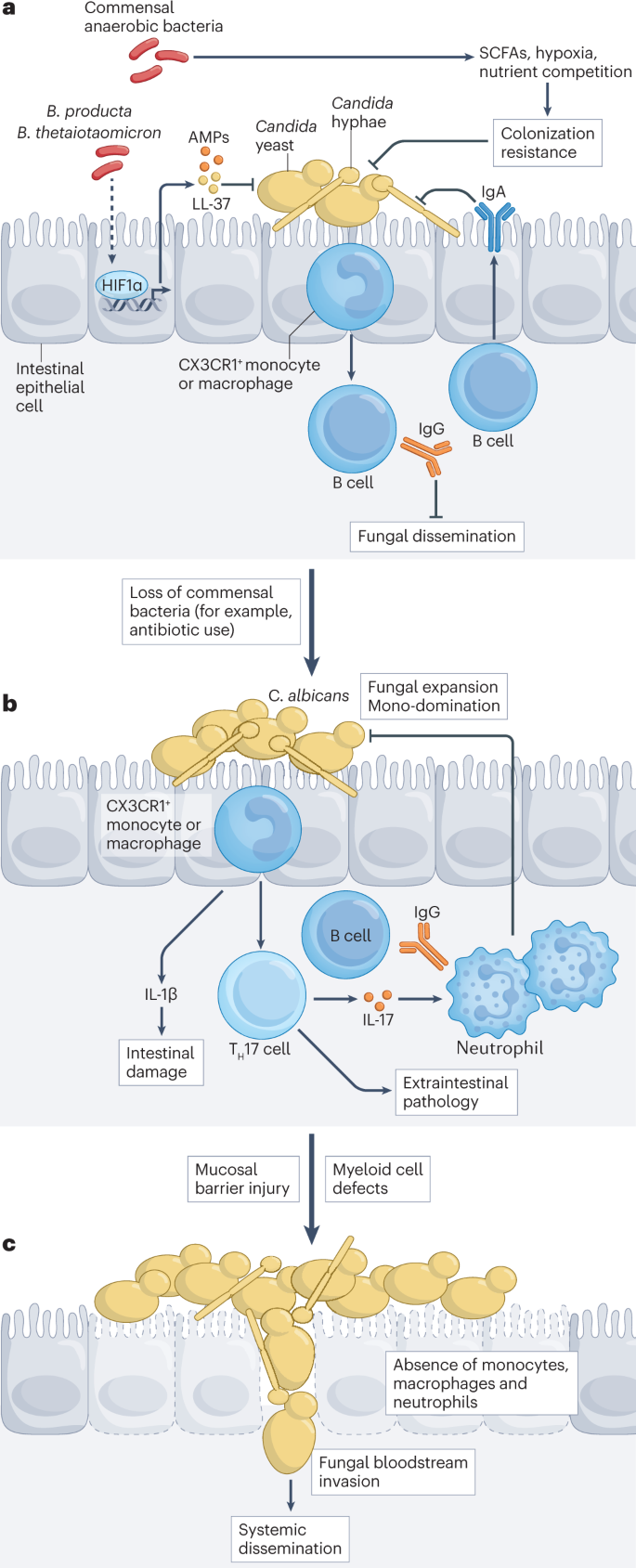
a , Mucosal and intestinal bacterial communities outnumber and compete with site-specific endogenous fungi. In the intestine, commensal anaerobic bacteria, exemplified by Blautia producta and Bacteroides thetaiotaomicron , provide colonization resistance against Candida species by activating the transcription factor hypoxia-inducible factor 1α (HIF1α) in intestinal epithelial cells to regulate the release of LL-37, an antimicrobial peptide (AMP) that restricts Candida albicans growth. Bacterial products of fermentation, specifically short-chain fatty acids (SCFAs), and metabolites likely also contribute to fungal colonization resistance and inhibition of Candida filamentation. Nutrient competition and regulation of tissue oxygen levels contribute to the balance between endogenous bacteria and competing fungal species in the gastrointestinal and reproductive tracts. CX3CR1 + mononuclear phagocytes recognize intestinal fungi through C-type lectin receptor (CLR)–CARD9 signalling and regulate the production of IgA and IgG antibodies to Candida by B cells. IgA antibodies recognize and target C. albicans adhesins (such as agglutinin-like sequence 3 (Als3)) that are primarily expressed by pseudohyphae and thus promote maintenance of the commensal yeast cell morphology. Candida -targeted IgG antibodies can protect against bloodstream invasion and disseminated candidiasis. b , In the intestinal and reproductive tracts, antibiotic-induced loss of predominantly anaerobic bacterial taxa reduces Candida colonization resistance and facilitates domination by individual fungal taxa. C. albicans strains from patients with inflammatory bowel disease with fungal dysbiosis can aggravate tissue damage, correlating with fungal strain-specific induction of IL-1β production by immune cells. The induction of T helper 17 (T H 17) cells and IL-17 production can promote neutrophil recruitment to inhibit fungal tissue invasion and dissemination. However, Candida -specific T H 17 cells can also promote extraintestinal inflammation. For example, mould aeroallergens can stimulate C. albicans -primed T H 17 cells and aggravate fungus-associated asthma. c , Systemic bloodstream infections can arise from intestinal fungal dysbiosis with concomitant epithelial cell damage (for example, following chemotherapy) and with defects in the number or function of circulating myeloid phagocytes.
In early human life, fungal–bacterial interactions shape the orderly assembly of the microbiota 158 . For example, ecological modelling in preterm neonates in an intensive care unit predicted that high abundance of intestinal Candida inhibited the expansion of Enterobacteriaceae, which facilitate the ensuing transition to commensal bacterial anaerobes 159 . This prediction was validated in interkingdom co-culture experiments with infant-derived Candida strains and in sequential intestinal colonization studies in mice, which showed that gut-resident Candida decreased the load of sequentially introduced Enterobacteriaceae. A recent review provides an excellent summary of numerous studies on the functional interactions between commensal, probiotic and potentially pathogenic bacteria and fungi 160 .
In addition to the role of fungi in microbiota assembly, the density of intestinal fungi and, in particular, the abundance of Pichia kudriavzevii (the sexual state of Candida krusei ) have been linked to subsequent atopy in independent birth cohorts 161 . Transient P. kudriavzevii colonization exacerbated house dust mite-induced allergic airway disease in neonatal specific pathogen-free mice 161 . In human adults, circulating fungus-reactive memory T H 17 cells preferentially target C. albicans -derived antigens, consistent with the idea that intestinal colonization with C. albicans induces T H 17 cell differentiation 162 . Circulating C. albicans -induced T H 17 cells cross-react with other fungi and can exacerbate the pathology of A. fumigatus -triggered allergic lung disease.
Malassezia , the major fungal commensal of human skin 163 , can cause pityriasis versicolor, characterized by hyperpigmented or hypopigmented flaky skin lesions. In a mouse epicutaneous infection model, Malassezia induced T H 17 cell differentiation and elicited IL-23-dependent IL-17 release by γδ T cells and type 3 innate lymphoid cells 164 . These sources of IL-17 restrict fungal growth in intact skin but can exacerbate Malassezia -driven inflammation in injured skin. Local IL-17 recall responses to Malassezia and Candida also aggravated psoriasiform skin inflammation in mice, which recapitulates transcriptional features of human psoriatic skin lesions 165 .
In the gut, the humoral immune response can shape mutualism between intestinal Candida and its human host by preferentially targeting adhesins expressed by C. albicans hyphae but not the yeast form. This IgA-dependent selection favours the growth of yeast rather than hyphal morphotypes in the intestine and enhances the competitive fitness of yeast in antibiotic-treated mice 166 , 167 . Furthermore, the human repertoire of antibodies to C. albicans includes IgG antibodies, which depend on CARD9 + intestinal macrophages to promote their production and are protective in mice infected systemically with C. albicans and C. auris 168 .
Commensal anaerobic bacteria can regulate intestinal fungal density, partly by inducing the production of antimicrobial peptides, by producing short-chain fatty acids through fermenting dietary fibre and by maintaining a low-oxygen environment. Bacteroides and Blautia bacteria activate intestinal epithelial cell signalling through HIF1α, which regulates enterocyte release of the antimicrobial peptide LL-37 and C. albicans colonization resistance 43 (Fig. 4a ). Antibiotics that promote the loss of anaerobic bacterial taxa drive intestinal fungal expansion. For example, antibiotic-induced injury to the bacterial microbiota facilitates intestinal expansion and domination by pathogenic Candida (Fig. 4b ), which in the context of chemotherapy-induced mucosal injury can translocate across the intestinal barrier to cause life-threatening bloodstream infections in HSCT recipients 169 (Fig. 4c ). Intestinal fungal dysbiosis during the peri-HSCT period is also linked to reduced long-term survival and increased non-relapse mortality 170 , 171 .
In human cohorts, fungal gut dysbiosis characterized by Malassezia , Debaromyces or Candida expansion is associated with inflammatory bowel disease (IBD) phenotypes. In mice, during intestinal wound healing and dextran sodium sulfate (DSS)-induced colitis, the introduction of representative clinical strains from individual fungal genera ( Malassezia , Debaromyces or Candida ) caused exuberant tissue pathology, whereas laboratory-passaged strains did not 172 , 173 , 174 , 175 . Genetic variation in CLEC7A (Y238*) and CARD9 (S12N) is associated with increased risk and severity of IBD 157 , 176 , 177 . Responsiveness to faecal microbiota transplantation in patients with IBD is associated with high levels of Candida before faecal microbiota transplantation, and disease amelioration is associated with low levels of Candida after faecal microbiota transplantation, which is consistent with the idea that faecal microbiota transplantation may reduce the contribution of fungus-associated inflammation to IBD severity 178 .
A recent study explored how fungal strain-specific attributes affect functional interactions with host cells to affect IBD severity. A collection of C. albicans strains cultured from colonic lavages of patients with IBD and non-disease controls was classified on the basis of filamentation properties and ability to induce IL-1β production by macrophages as a measure of strain-dependent aggravation of intestinal inflammation 179 (Fig. 4b ). High damage-inducing strains induced IL-1β-dependent tissue damage and instructed T H 17 cell differentiation through recognition of candidalysin. These strain attributes were linked to IBD severity in humans and were recapitulated in a mouse DSS-induced colitis model, in which IL-1 blockade ameliorated inflammation.
In the pancreas, tissue-resident fungi (particularly Malassezia ) are markedly enriched in pancreatic ductal adenocarcinoma compared with non-cancerous tissue 180 . In a mouse model of pancreatic ductal adenocarcinoma, antifungal drugs ameliorated tumour progression and the reintroduction of Malassezia accelerated tumour growth. Mechanistically, mannose-binding lectin bound to Malassezia glycans and activated the C3 complement cascade, which enhanced the growth of tumour cells expressing C3a receptor 180 . Depletion of commensal fungi was recently shown to enhance responsiveness to radiotherapy in a mouse model of breast cancer, and intratumoural dectin 1 expression negatively correlated with survival of patients with breast cancer 181 . The recent comprehensive characterization of the composition of the tumour-associated mycobiota across multiple human cancers has revealed the low-level, yet ubiquitous, presence of fungal DNA and cells in a tumour type-specific manner, often in close spatial association with macrophages and malignant cells 182 , 183 . Clinically, certain plasma or tumour-associated mycobiota subtypes were associated with differential response to immunotherapy, disease severity and/or survival, which indicates that cell-free or tumour-associated fungal DNA may be a potentially promising diagnostic and prognostic biomarker in patients with cancer 182 , 183 .
Box 3 The human mycobiota: localization, species and biomass
Fungi are core constituents of microbial communities in human tissues, including the skin, mouth and respiratory, intestinal and genitourinary tracts 160 , although they typically account for less than 1% of total microbial amplicon sequences, with 50–100 fungal genera being reliably identified 202 . A core fungal signature at different tissue sites has been challenging to define, as humans inhale and ingest fungi daily, blurring the distinction between passenger and colonizer species. Longitudinally collected faecal samples show high intra-individual and interindividual variability of fungal taxa, and low day-to-day stability of intestinal fungi, which is in contrast to the relative intra-individual stability of bacterial communities 170 , 203 . Frequent tooth brushing reduced Candida levels in dental plaque, saliva and stool, which is consistent with the oral reservoir contributing to intestinal flux 204 . Saccharomyces stool levels dropped precipitously when human volunteers consumed Saccharomyces -free diets, only to increase again when the volunteers ate a single bite of bread 204 . Notwithstanding this variability, certain genera predominate at distinct sites: Saccharomyces , Candida , Debaromyces and Pichia are commonly found in the digestive and genital tracts, Malassezia is commonly found in the skin, and Aspergillus , Fusarium , Alternaria and Cladosporium moulds are commonly found in the airways, albeit at lower densities. The figure depicts commensal fungal communities that are adapted to specific tissue niches of humans. Common taxa found under homeostatic conditions are indicated, as are common diseases associated with some of these taxa, such as invasive candidiasis, vulvovaginal candidiasis (VVC) and pityriasis versicolor.
Nucleic acid-based methods underestimate fungal biomass given the size difference of bacteria (~0.5–2-μm diameter) and fungi (~3–5-μm diameter for yeasts and ~10 to >100 μm and greater diameter for hyphae) and metagenomic sequencing has not been widely applied to analyse fungal communities, owing to large and complex fungal genomes (typically more than 20 Mb in size, with both haploid and diploid genomes), low fungal reads compared with bacteria and incomplete, poorly annotated reference libraries 202 . Thus, the true size and composition of the mycobiome in humans remain incompletely understood.
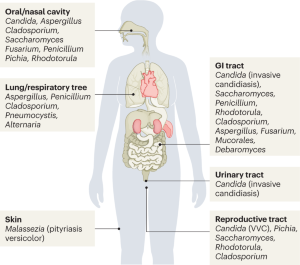
Conclusions and future perspectives
Opportunistic fungal infections cause a substantial disease burden globally and are a major threat to the well-being of vulnerable patients. The past decade of research on fungal immunology has moved at a remarkable pace and has yielded exciting advances in our basic understanding of the cellular and molecular determinants of antifungal defences. In this Review, we have delineated promising immunogenomic factors that may enable personalized risk assessment and prognosis of patients at risk of fungal disease, and adjunctive immunotherapeutic strategies that may protect fungus-infected patients by modulating antifungal responses. We have highlighted the expanding universe of inherited and iatrogenic immunodeficiency conditions that predispose to opportunistic fungal disease, as well as the conceptual advances in our understanding of protective and pathogenic antifungal immune pathways that have been derived from studying these conditions. We have also discussed how the endogenous mycobiota shape immune homeostasis and regulate responses to neoplasia, infections, autoimmunity and other exogenous insults. Moving forward, further increasing our understanding of tissue-specific mechanisms of antifungal defence should help to improve the management of life-threatening fungal infections and curtail their detrimental impact on human health.
Hoenigl, M. et al. COVID-19-associated fungal infections. Nat. Microbiol. 7 , 1127–1140 (2022).
Article CAS PubMed PubMed Central Google Scholar
Lionakis, M. S. & Hohl, T. M. Call to action: how to tackle emerging nosocomial fungal infections. Cell Host Microbe 27 , 859–862 (2020).
Salazar, F., Bignell, E., Brown, G. D., Cook, P. C. & Warris, A. Pathogenesis of respiratory viral and fungal coinfections. Clin. Microbiol. Rev. 35 , e0009421 (2022).
Article PubMed Google Scholar
Chow, N. A. et al. Multiple introductions and subsequent transmission of multidrug-resistant Candida auris in the USA: a molecular epidemiological survey. Lancet Infect. Dis. 18 , 1377–1384 (2018).
Article PubMed PubMed Central Google Scholar
Brown, G. D., Willment, J. A. & Whitehead, L. C-type lectins in immunity and homeostasis. Nat. Rev. Immunol. 18 , 374–389 (2018). This review provides a comprehensive overview of the role of C-type lectins in immune homeostasis and host defence .
Article CAS PubMed Google Scholar
Borriello, F. et al. An adjuvant strategy enabled by modulation of the physical properties of microbial ligands expands antigen immunogenicity. Cell 185 , 614–629 e621 (2022).
Corvilain, E., Casanova, J. L. & Puel, A. Inherited CARD9 deficiency: invasive disease caused by ascomycete fungi in previously healthy children and adults. J. Clin. Immunol. 38 , 656–693 (2018).
Pappas, P. G., Lionakis, M. S., Arendrup, M. C., Ostrosky-Zeichner, L. & Kullberg, B. J. Invasive candidiasis. Nat. Rev. Dis. Prim. 4 , 18026 (2018).
Lionakis, M. S. & Levitz, S. M. Host control of fungal infections: lessons from basic studies and human cohorts. Annu. Rev. Immunol. 36 , 157–191 (2018).
Gaffen, S. L. & Moutsopoulos, N. M. Regulation of host-microbe interactions at oral mucosal barriers by type 17 immunity. Sci. Immunol. 5 , eaau4594 (2020).
Puel, A. et al. Chronic mucocutaneous candidiasis in humans with inborn errors of interleukin-17 immunity. Science 332 , 65–68 (2011). This is the first study showing that impaired IL-17 responses underlie chronic mucocutaneous candidiasis in humans .
Boisson, B. et al. An ACT1 mutation selectively abolishes interleukin-17 responses in humans with chronic mucocutaneous candidiasis. Immunity 39 , 676–686 (2013).
Conti, H. R. et al. IL-17 receptor signaling in oral epithelial cells is critical for protection against oropharyngeal candidiasis. Cell Host Microbe 20 , 606–617 (2016).
Conti, H. R. et al. Oral-resident natural Th17 cells and gammadelta T cells control opportunistic Candida albicans infections. J. Exp. Med. 211 , 2075–2084 (2014). This study reveals the crucial contribution of both natural T H 17 cells and γδ T cells in controlling Candida in the oral mucosa .
Conti, H. R. et al. Th17 cells and IL-17 receptor signaling are essential for mucosal host defense against oral candidiasis. J. Exp. Med. 206 , 299–311 (2009). This is the first study to show the crucial contribution of type 17 immune responses in mucosal host defence against Candida in mice .
Levy, R. et al. Genetic, immunological, and clinical features of patients with bacterial and fungal infections due to inherited IL-17RA deficiency. Proc. Natl Acad. Sci. Usa. 113 , E8277–E8285 (2016).
Puel, A. et al. Inborn errors of human IL-17 immunity underlie chronic mucocutaneous candidiasis. Curr. Opin. Allergy Clin. Immunol. 12 , 616–622 (2012).
Oikonomou, V., Break, T. J., Gaffen, S. L., Moutsopoulos, N. M. & Lionakis, M. S. Infections in the monogenic autoimmune syndrome APECED. Curr. Opin. Immunol. 72 , 286–297 (2021).
Davidson, L. et al. Risk of candidiasis associated with interleukin-17 inhibitors: a real-world observational study of multiple independent sources. Lancet Reg. Health Eur. 13 , 100266 (2022).
Swidergall, M. & LeibundGut-Landmann, S. Immunosurveillance of Candida albicans commensalism by the adaptive immune system. Mucosal Immunol. 15 , 829–836 (2022).
Aggor, F. E. et al. A gut-oral microbiome-driven axis controls oropharyngeal candidiasis through retinoic acid. JCI Insight 7 , e160348 (2022).
Verma, A. H. et al. Oral epithelial cells orchestrate innate type 17 responses to Candida albicans through the virulence factor candidalysin. Sci. Immunol. 2 , eaam8834 (2017).
Basso, P. et al. Deep tissue infection by an invasive human fungal pathogen requires lipid-based suppression of the IL-17 response. Cell Host Microbe 30 , 1589–1601 e1585 (2022).
Lisco, A. et al. Lost in translation: lack of CD4 expression due to a novel genetic defect. J. Infect. Dis. 223 , 645–654 (2021).
Cudrici, C. D. et al. Characterization of autoantibodies, immunophenotype and autoimmune disease in a prospective cohort of patients with idiopathic CD4 lymphocytopenia. Clin. Immunol. 224 , 108664 (2021).
Hernandez-Santos, N. et al. Th17 cells confer long-term adaptive immunity to oral mucosal Candida albicans infections. Mucosal Immunol. 6 , 900–910 (2013).
Swidergall, M., Solis, N. V., Lionakis, M. S. & Filler, S. G. EphA2 is an epithelial cell pattern recognition receptor for fungal beta-glucans. Nat. Microbiol. 3 , 53–61 (2018).
Aggor, F. E. Y. et al. Oral epithelial IL-22/STAT3 signaling licenses IL-17-mediated immunity to oral mucosal candidiasis. Sci. Immunol. 5 , eaba0570 (2020). This study defines the mechanistic role of the type 17 cytokine IL-22 in promoting protection during mucosal candidiasis in mice .
Puel, A. et al. Autoantibodies against IL-17A, IL-17F, and IL-22 in patients with chronic mucocutaneous candidiasis and autoimmune polyendocrine syndrome type I. J. Exp. Med. 207 , 291–297 (2010).
Kisand, K. et al. Chronic mucocutaneous candidiasis in APECED or thymoma patients correlates with autoimmunity to Th17-associated cytokines. J. Exp. Med. 207 , 299–308 (2010).
Break, T. J. et al. Aberrant type 1 immunity drives susceptibility to mucosal fungal infections. Science 371 , eaay5731 (2021). This study uncovers the contribution of exacerbated mucosal type 1 immune responses in promoting mucosal fungal infection by disrupting the integrity of the epithelial barrier .
Toubiana, J. et al. Heterozygous STAT1 gain-of-function mutations underlie an unexpectedly broad clinical phenotype. Blood 127 , 3154–3164 (2016).
Yano, J. et al. Current patient perspectives of vulvovaginal candidiasis: incidence, symptoms, management and post-treatment outcomes. BMC Women’s Health 19 , 48 (2019).
Lopes, J. P. & Lionakis, M. S. Pathogenesis and virulence of Candida albicans. Virulence 13 , 89–121 (2022).
Peters, B. M. et al. The interleukin (IL) 17R/IL-22R signaling axis is dispensable for Vulvovaginal candidiasis regardless of estrogen status. J. Infect. Dis. 221 , 1554–1563 (2020).
MacAlpine, J. et al. A small molecule produced by Lactobacillus species blocks Candida albicans filamentation by inhibiting a DYRK1-family kinase. Nat. Commun. 12 , 6151 (2021).
Edwards, J. E. Jr. et al. A fungal immunotherapeutic vaccine (NDV-3A) for treatment of recurrent vulvovaginal candidiasis-a phase 2 randomized, double-blind, placebo-controlled trial. Clin. Infect. Dis. 66 , 1928–1936 (2018).
Ibrahim, A. S. et al. NDV-3 protects mice from vulvovaginal candidiasis through T- and B-cell immune response. Vaccine 31 , 5549–5556 (2013).
Kashem, S. W. et al. Nociceptive sensory fibers drive interleukin-23 production from CD301b + dermal dendritic cells and drive protective cutaneous immunity. Immunity 43 , 515–526 (2015).
Cohen, J. A. et al. Cutaneous TRPV1 + neurons trigger protective innate type 17 anticipatory immunity. Cell 178 , 919–932 e914 (2019). This study shows the importance of TRPV1 + sensory neuron activation in mediating protective type 17 immune responses in Candida -infected skin .
Leonardi, I. et al. Mucosal fungi promote gut barrier function and social behavior via type 17 immunity. Cell 185 , 831–846 e814 (2022).
Huh, J. R. & Veiga-Fernandes, H. Neuroimmune circuits in inter-organ communication. Nat. Rev. Immunol. 20 , 217–228 (2020).
Fan, D. et al. Activation of HIF-1alpha and LL-37 by commensal bacteria inhibits Candida albicans colonization. Nat. Med. 21 , 808–814 (2015). This study uncovers the molecular basis of colonization resistance conferred by certain commensal bacterial communities against Candida albicans in the gut .
Drummond, R. A. et al. Long-term antibiotic exposure promotes mortality after systemic fungal infection by driving lymphocyte dysfunction and systemic escape of commensal bacteria. Cell Host Microbe 30 , 1020–1033.e6 (2022). This study shows that antibiotic exposure increases susceptibility to mouse invasive candidiasis by impairing lymphocyte IL-17A and GM-CSF responses in the gut .
Glocker, E. O. et al. A homozygous CARD9 mutation in a family with susceptibility to fungal infections. N. Engl. J. Med. 361 , 1727–1735 (2009). This is the first study to show that human CARD9 deficiency underlies susceptibility to mucosal and invasive fungal disease .
Drummond, R. A. et al. CARD9 + microglia promote antifungal immunity via IL-1β - and CXCL1-mediated neutrophil recruitment. Nat. Immunol. 20 , 559–570 (2019). This study defines the mechanisms by which CARD9-dependent microglia–neutrophil crosstalk promotes protective immunity in the Candida -infected brain .
Jodele, S. et al. Complement blockade for TA-TMA: lessons learned from a large pediatric cohort treated with eculizumab. Blood 135 , 1049–1057 (2020).
PubMed PubMed Central Google Scholar
Bar, E., Whitney, P. G., Moor, K., Reis e Sousa, C. & LeibundGut-Landmann, S. IL-17 regulates systemic fungal immunity by controlling the functional competence of NK cells. Immunity 40 , 117–127 (2014).
Dominguez-Andres, J. et al. Inflammatory Ly6Chigh monocytes protect against candidiasis through IL-15-driven NK cell/neutrophil activation. Immunity 46 , 1059–1072 e1054 (2017).
Wan, L. et al. Effect of granulocyte-macrophage colony-stimulating factor on prevention and treatment of invasive fungal disease in recipients of allogeneic stem-cell transplantation: a prospective multicenter randomized phase IV trial. J. Clin. Oncol. 33 , 3999–4006 (2015).
Li, D. D. et al. Fungal sensing enhances neutrophil metabolic fitness by regulating antifungal Glut1 activity. Cell Host Microbe 30 , 530–544 e536 (2022).
Jawale, C. V. et al. Restoring glucose uptake rescues neutrophil dysfunction and protects against systemic fungal infection in mouse models of kidney disease. Sci. Transl. Med. 12 , eaay5691 (2020).
Candon, S. et al. Chronic disseminated candidiasis during hematological malignancies: an immune reconstitution inflammatory syndrome with expansion of pathogen-specific T helper type 1 cells. J. Infect. Dis. 221 , 1907–1916 (2020).
Garg, A. V. et al. MCPIP1 endoribonuclease activity negatively regulates interleukin-17-mediated signaling and inflammation. Immunity 43 , 475–487 (2015).
Lionakis, M. S. et al. Chemokine receptor Ccr1 drives neutrophil-mediated kidney immunopathology and mortality in invasive candidiasis. PLoS Pathog. 8 , e1002865 (2012).
Del Fresno, C. et al. DNGR-1 in dendritic cells limits tissue damage by dampening neutrophil recruitment. Science 362 , 351–356 (2018).
Liu, J. et al. Progranulin aggravates lethal Candida albicans sepsis by regulating inflammatory response and antifungal immunity. PLoS Pathog. 18 , e1010873 (2022).
Ramani, K. et al. The kallikrein-kinin system: a novel mediator of IL-17-driven anti-Candida immunity in the kidney. PLoS Pathog. 12 , e1005952 (2016).
Lionakis, M. S. et al. CX3CR1-dependent renal macrophage survival promotes Candida control and host survival. J. Clin. Invest. 123 , 5035–5051 (2013).
Ngo, L. Y. et al. Inflammatory monocytes mediate early and organ-specific innate defense during systemic candidiasis. J. Infect. Dis. 209 , 109–119 (2014).
Cheng, S. C. et al. mTOR- and HIF-1alpha-mediated aerobic glycolysis as metabolic basis for trained immunity. Science 345 , 1250684 (2014). This study defines the metabolic and epigenetic alterations that underlie the development of protective trained immunity to candidiasis .
Zhao, X. et al. JNK1 negatively controls antifungal innate immunity by suppressing CD23 expression. Nat. Med. 23 , 337–346 (2017).
Li, J. et al. Chronic mucocutaneous candidiasis and connective tissue disorder in humans with impaired JNK1-dependent responses to IL-17A/F and TGF-beta. Sci. Immunol. 4 , eaax7965 (2019).
Fitzpatrick, Z. et al. Gut-educated IgA plasma cells defend the meningeal venous sinuses. Nature 587 , 472–476 (2020).
Kumar, V. et al. Immunochip SNP array identifies novel genetic variants conferring susceptibility to candidaemia. Nat. Commun. 5 , 4675 (2014).
Latge, J. P. & Chamilos, G. Aspergillus fumigatus and aspergillosis in 2019. Clin. Microbiol. Rev. 33 , e00140-18 (2019).
Espinosa, V. et al. Inflammatory monocytes orchestrate innate antifungal immunity in the lung. PLoS Pathog. 10 , e1003940 (2014).
Schmidt, S. et al. Human natural killer cells exhibit direct activity against Aspergillus fumigatus hyphae, but not against resting conidia. J. Infect. Dis. 203 , 430–435 (2011).
Seif, M. et al. CAR T cells targeting Aspergillus fumigatus are effective at treating invasive pulmonary aspergillosis in preclinical models. Sci. Transl. Med. 14 , eabh1209 (2022).
Cadena, J., Thompson, G. R. III & Patterson, T. F. Aspergillosis: epidemiology, diagnosis, and treatment. Infect. Dis. Clin. North. Am. 35 , 415–434 (2021).
Agarwal, R. et al. Allergic bronchopulmonary aspergillosis. Clin. Chest Med. 43 , 99–125 (2022).
Philippe, B. et al. Killing of Aspergillus fumigatus by alveolar macrophages is mediated by reactive oxidant intermediates. Infect. Immun. 71 , 3034–3042 (2003).
Hohl, T. M. et al. Aspergillus fumigatus triggers inflammatory responses by stage-specific beta-glucan display. PLoS Pathog. 1 , e30 (2005).
Aimanianda, V. et al. Surface hydrophobin prevents immune recognition of airborne fungal spores. Nature 460 , 1117–1121 (2009).
Doni, A. et al. Serum amyloid P component is an essential element of resistance against Aspergillus fumigatus. Nat. Commun. 12 , 3739 (2021).
Garlanda, C. et al. Non-redundant role of the long pentraxin PTX3 in anti-fungal innate immune response. Nature 420 , 182–186 (2002).
Stappers, M. H. T. et al. Recognition of DHN-melanin by a C-type lectin receptor is required for immunity to Aspergillus. Nature 555 , 382–386 (2018).
Liu, H. et al. Aspergillus fumigatus CalA binds to integrin alpha5beta1 and mediates host cell invasion. Nat. Microbiol. 2 , 16211 (2016).
Leal, S. M. Jr. et al. Fungal antioxidant pathways promote survival against neutrophils during infection. J. Clin. Invest. 122 , 2482–2498 (2012).
Shlezinger, N. et al. Sterilizing immunity in the lung relies on targeting fungal apoptosis-like programmed cell death. Science 357 , 1037–1041 (2017). This study shows that neutrophils trigger a programmed cell death pathway with apoptosis-like features in Aspergillus conidia that is opposed by the fungal antiapoptotic protein AfBIR1 .
International Chronic Granulomatous Disease Cooperative Study Group. A controlled trial of interferon gamma to prevent infection in chronic granulomatous disease. N. Engl. J. Med. 324 , 509–516 (1991).
Article Google Scholar
Akoumianaki, T. et al. Aspergillus cell wall melanin blocks LC3-associated phagocytosis to promote pathogenicity. Cell Host Microbe 19 , 79–90 (2016).
Goncalves, S. M. et al. Phagosomal removal of fungal melanin reprograms macrophage metabolism to promote antifungal immunity. Nat. Commun. 11 , 2282 (2020).
Guo, Y. et al. During aspergillus infection, monocyte-derived DCs, neutrophils, and plasmacytoid DCs enhance innate immune defense through CXCR3-dependent crosstalk. Cell Host Microbe 28 , 104–116 e104 (2020). This study uncovers the intricate crosstalk between CXCR3 + plasmacytoid DCs, monocyte-derived DCs and neutrophils in orchestrating defence against Aspergillus in the lung .
Jhingran, A. et al. Compartment-specific and sequential role of MyD88 and CARD9 in chemokine induction and innate defense during respiratory fungal infection. PLoS Pathog. 11 , e1004589 (2015). This work defines the sequential roles of epithelial cell MYD88 and haematopoietic cell CARD9 signalling in driving protective neutrophil recruitment in the Aspergillus -infected lung .
Caffrey, A. K. et al. IL-1alpha signaling is critical for leukocyte recruitment after pulmonary Aspergillus fumigatus challenge. PLoS Pathog. 11 , e1004625 (2015).
Rieber, N. et al. Extrapulmonary Aspergillus infection in patients with CARD9 deficiency. JCI Insight 1 , e89890 (2016).
Wang, X. et al. MDA5 is an essential sensor of a pathogen-associated molecular pattern associated with vitality that is necessary for host resistance against Aspergillus fumigatus. J. Immunol. 205 , 3058–3070 (2020).
Caffrey-Carr, A. K. et al. Host-derived leukotriene B4 is critical for resistance against invasive pulmonary aspergillosis. Front. Immunol. 8 , 1984 (2017).
Snarr, B. D. et al. Galectin-3 enhances neutrophil motility and extravasation into the airways during Aspergillus fumigatus infection. PLoS Pathog. 16 , e1008741 (2020).
Hohl, T. M. et al. Inflammatory monocytes facilitate adaptive CD4 T cell responses during respiratory fungal infection. Cell Host Microbe 6 , 470–481 (2009).
Espinosa, V. et al. Type III interferon is a critical regulator of innate antifungal immunity. Sci. Immunol. 2 , eaan5357 (2017). This work uncovers the crucial contribution of type III interferon signalling in promoting neutrophil-dependent protection against pulmonary aspergillosis .
Espinosa, V. et al. Cutting edge: neutrophils license the maturation of monocytes into effective antifungal effectors. J. Immunol. 209 , 1827–1831 (2022).
Kasahara, S. et al. Role of granulocyte-macrophage colony-stimulating factor signaling in regulating neutrophil antifungal activity and the oxidative burst during respiratory fungal challenge. J. Infect. Dis. 213 , 1289–1298 (2016).
Punatar, A. D., Kusne, S., Blair, J. E., Seville, M. T. & Vikram, H. R. Opportunistic infections in patients with pulmonary alveolar proteinosis. J. Infect. 65 , 173–179 (2012).
Zarember, K. A., Sugui, J. A., Chang, Y. C., Kwon-Chung, K. J. & Gallin, J. I. Human polymorphonuclear leukocytes inhibit Aspergillus fumigatus conidial growth by lactoferrin-mediated iron depletion. J. Immunol. 178 , 6367–6373 (2007).
Clark, H. L. et al. Zinc and manganese chelation by neutrophil S100A8/A9 (calprotectin) limits extracellular aspergillus fumigatus hyphal growth and corneal infection. J. Immunol. 196 , 336–344 (2016).
Cunha, C. et al. Genetic PTX3 deficiency and aspergillosis in stem-cell transplantation. N. Engl. J. Med. 370 , 421–432 (2014). This study shows that genetic variation at the PTX3 locus impairs fungal uptake and killing by neutrophils and contributes to susceptibility to aspergillosis in HSCT recipients .
Fisher, C. E. et al. Validation of single nucleotide polymorphisms in invasive aspergillosis following hematopoietic cell transplantation. Blood 129 , 2693–2701 (2017).
Lionakis, M. S. et al. Inhibition of B cell receptor signaling by ibrutinib in primary CNS lymphoma. Cancer Cell 31 , 833–843 e835 (2017).
Ghez, D. et al. Early-onset invasive aspergillosis and other fungal infections in patients treated with ibrutinib. Blood 131 , 1955–1959 (2018).
Herbst, S. et al. Phagocytosis-dependent activation of a TLR9-BTK-calcineurin-NFAT pathway co-ordinates innate immunity to Aspergillus fumigatus. EMBO Mol. Med. 7 , 240–258 (2015).
Roden, M. M. et al. Epidemiology and outcome of zygomycosis: a review of 929 reported cases. Clin. Infect. Dis. 41 , 634–653 (2005).
Soliman, S. S. M. et al. Mucoricin is a ricin-like toxin that is critical for the pathogenesis of mucormycosis. Nat. Microbiol. 6 , 313–326 (2021).
Liu, M. et al. The endothelial cell receptor GRP78 is required for mucormycosis pathogenesis in diabetic mice. J. Clin. Invest. 120 , 1914–1924 (2010).
Gebremariam, T. et al. CotH3 mediates fungal invasion of host cells during mucormycosis. J. Clin. Invest. 124 , 237–250 (2014). Liu et al. (2010) and Gebremariam et al. (2014) uncover the interplay between the Mucorales invasin CotH3 and the endothelial cell receptor GRP78 in promoting susceptibility to mucormycosis during diabetic ketoacidosis .
Gebremariam, T. et al. Bicarbonate correction of ketoacidosis alters host-pathogen interactions and alleviates mucormycosis. J. Clin. Invest. 126 , 2280–2294 (2016).
Alqarihi, A. et al. GRP78 and integrins play different roles in host cell invasion during mucormycosis. mBio 11 , e01087-20 (2020).
Watkins, T. N. et al. Inhibition of EGFR signaling protects from mucormycosis. mBio 9 , e01384-18 (2018).
Andrianaki, A. M. et al. Iron restriction inside macrophages regulates pulmonary host defense against Rhizopus species. Nat. Commun. 9 , 3333 (2018).
Siqueira, I. M. et al. Early immune response against Fonsecaea pedrosoi requires dectin-2-mediated Th17 activity, whereas Th1 response, aided by Treg cells, is crucial for fungal clearance in later stage of experimental chromoblastomycosis. PLoS Negl. Trop. Dis. 14 , e0008386 (2020).
Teixeira de Sousa Mda, G., Ghosn, E. E. & Almeida, S. R. Absence of CD4 + T cells impairs host defence of mice infected with Fonsecaea pedrosoi. Scand. J. Immunol. 64 , 595–600 (2006).
Sousa Mda, G. et al. Restoration of pattern recognition receptor costimulation to treat chromoblastomycosis, a chronic fungal infection of the skin. Cell Host Microbe 9 , 436–443 (2011).
de Sousa Mda, G. et al. Topical application of imiquimod as a treatment for chromoblastomycosis. Clin. Infect. Dis. 58 , 1734–1737 (2014).
Revankar, S. G., Patterson, J. E., Sutton, D. A., Pullen, R. & Rinaldi, M. G. Disseminated phaeohyphomycosis: review of an emerging mycosis. Clin. Infect. Dis. 34 , 467–476 (2002).
Kainer, M. A. et al. Fungal infections associated with contaminated methylprednisolone in Tennessee. N. Engl. J. Med. 367 , 2194–2203 (2012).
Drummond, R. A. et al. Human dectin-1 deficiency impairs macrophage-mediated defense against phaeohyphomycosis. J. Clin. Invest. 132 , e159348 (2022). This study shows that dectin 1 and CARD9 promote macrophage-dependent, IL-1β-mediated and TNF-mediated protection against melanin-bearing phaeohyphomycetes fungi, and that human dectin 1 deficiency may contribute to severe phaeohyphomycosis in ~70% of affected patients .
Mazzurco, J. D., Ramirez, J. & Fivenson, D. P. Phaeohyphomycosis caused by Phaeoacremonium species in a patient taking infliximab. J. Am. Acad. Dermatol. 66 , 333–335 (2012).
Casadevall, A. Immunity to invasive fungal diseases. Annu. Rev. Immunol. 40 , 121–141 (2022).
Erwig, L. P. & Gow, N. A. Interactions of fungal pathogens with phagocytes. Nat. Rev. Microbiol. 14 , 163–176 (2016).
Browne, S. K. et al. Adult-onset immunodeficiency in Thailand and Taiwan. N. Engl. J. Med. 367 , 725–734 (2012).
Browne, S. K. et al. Anti-CD20 (rituximab) therapy for anti-IFN-gamma autoantibody-associated nontuberculous mycobacterial infection. Blood 119 , 3933–3939 (2012).
Ochoa, S. et al. Daratumumab (anti-CD38) for treatment of disseminated nontuberculous mycobacteria in a patient with anti-interferon-gamma autoantibodies. Clin. Infect. Dis. 72 , 2206–2208 (2021).
Francoise, U., Lafont, E., Suarez, F., Lanternier, F. & Lortholary, O. Disseminated cryptococcosis in a patient with CD40 ligand deficiency. J. Clin. Immunol. 42 , 1622–1625 (2022).
Vinh, D. C. et al. Autosomal dominant and sporadic monocytopenia with susceptibility to mycobacteria, fungi, papillomaviruses, and myelodysplasia. Blood 115 , 1519–1529 (2010).
Na, Y. R. et al. GM-CSF induces inflammatory macrophages by regulating glycolysis and lipid metabolism. J. Immunol. 197 , 4101 (2016).
Saijo, T. et al. Anti-granulocyte-macrophage colony-stimulating factor autoantibodies are a risk factor for central nervous system infection by Cryptococcus gattii in otherwise immunocompetent patients. mBio 5 , e00912-14 (2014).
Kannambath, S. et al. Genome-wide association study identifies novel colony stimulating factor 1 locus conferring susceptibility to cryptococcosis in human immunodeficiency virus-infected South Africans. Open Forum Infect. Dis. 7 , ofaa489 (2020).
Elmore, M. R. P. et al. Colony-stimulating factor 1 receptor signaling is necessary for microglia viability, unmasking a microglia progenitor cell in the adult brain. Neuron 82 , 380–397 (2014).
Bryan, A. M. et al. FTY720 reactivates cryptococcal granulomas in mice through S1P receptor 3 on macrophages. J. Clin. Invest. 130 , 4546–4560 (2020). This work uncovers the mechanisms by which the S1PR inhibitor fingolimod increases susceptibility to cryptococcosis by showing that it impairs S1PR3-mediated macrophage uptake and killing of fungi and granuloma formation .
Lee, S. C., Dickson, D. W. & Casadevall, A. Pathology of cryptococcal meningoencephalitis: analysis of 27 patients with pathogenetic implications. Hum. Pathol. 27 , 839–847 (1996).
Johnston, S. A. & May, R. C. The human fungal pathogen Cryptococcus neoformans escapes macrophages by a phagosome emptying mechanism that is inhibited by Arp2/3 complex-mediated actin polymerisation. PLoS Pathog. 6 , e1001041 (2010).
Fu, M. S. et al. Cryptococcus neoformans urease affects the outcome of intracellular pathogenesis by modulating phagolysosomal pH. PLoS Pathog. 14 , e1007144 (2018).
Scriven, J. E. et al. The CSF immune response in HIV-1-associated cryptococcal meningitis: macrophage activation, correlates of disease severity, and effect of antiretroviral therapy. J. Acquir. Immune Defic. Syndr. 75 , 299–307 (2017).
Jarvis, J. N. et al. Cerebrospinal fluid cytokine profiles predict risk of early mortality and immune reconstitution inflammatory syndrome in HIV-associated cryptococcal meningitis. PLoS Pathog. 11 , e1004754 (2015).
Jarvis, J. N. et al. The phenotype of the Cryptococcus -specific CD4 + memory T-cell response is associated with disease severity and outcome in HIV-associated cryptococcal meningitis. J. Infect. Dis. 207 , 1817–1828 (2013).
Jarvis, J. N. et al. Adjunctive interferon-γ immunotherapy for the treatment of HIV-associated cryptococcal meningitis: a randomized controlled trial. AIDS 26 , 1105–1113 (2012).
Anjum, S. et al. Outcomes in previously healthy cryptococcal meningoencephalitis patients treated with pulse taper corticosteroids for post-infectious inflammatory syndrome. Clin. Infect. Dis. 73 , e2789–e2798 (2021).
Panackal, A. A. et al. Paradoxical immune responses in non-HIV cryptococcal meningitis. PLoS Pathog. 11 , e1004884 (2015).
Xu, J. et al. Chemokine receptor CXCR3 is required for lethal brain pathology but not pathogen clearance during cryptococcal meningoencephalitis. Sci. Adv. 6 , eaba2502 (2020).
Antonelli, L. R. et al. Elevated frequencies of highly activated CD4 + T cells in HIV + patients developing immune reconstitution inflammatory syndrome. Blood 116 , 3818–3827 (2010). This work describes enhanced type 1 immune responses in patients with HIV/AIDS with IRIS .
Khaw, Y. M. et al. Th1-dependent cryptococcus-associated immune reconstitution inflammatory syndrome model with brain damage. Front. Immunol. 11 , 529219 (2020).
Hsu, A. P. et al. Immunogenetics associated with severe coccidioidomycosis. JCI Insight https://doi.org/10.1172/jci.insight.159491 (2022).
Wuthrich, M., Deepe, G. S. Jr & Klein, B. Adaptive immunity to fungi. Annu. Rev. Immunol. 30 , 115–148 (2012).
Subramanian Vignesh, K., Landero Figueroa, J. A., Porollo, A., Caruso, J. A. & Deepe, G. S. Jr. Granulocyte macrophage-colony stimulating factor induced Zn sequestration enhances macrophage superoxide and limits intracellular pathogen survival. Immunity 39 , 697–710 (2013).
Wuthrich, M., Ersland, K., Sullivan, T., Galles, K. & Klein, B. S. Fungi subvert vaccine T cell priming at the respiratory mucosa by preventing chemokine-induced influx of inflammatory monocytes. Immunity 36 , 680–692 (2012).
Tsai, M. et al. Disseminated coccidioidomycosis treated with interferon-gamma and dupilumab. N. Engl. J. Med. 382 , 2337–2343 (2020). This case report highlights the therapeutic benefit of pharmacological restoration of the T H 1 cell–T H 2 cell balance in coccidioidomycosis .
Vargas, S. L. et al. Search for primary infection by Pneumocystis carinii in a cohort of normal, healthy infants. Clin. Infect. Dis. 32 , 855–861 (2001).
Hoving, J. C. & Kolls, J. K. New advances in understanding the host immune response to Pneumocystis. Curr. Opin. Microbiol. 40 , 65–71 (2017).
Elsegeiny, W. et al. Murine models of Pneumocystis infection recapitulate human primary immune disorders. JCI Insight 3 , e91894 (2018). This study defines the IL-21R-dependent and STAT3-dependent mechanisms of protection by CD4 + T cells against Pneumocystis , thereby shedding light on the corresponding inborn errors of immunity that promote human susceptibility to the infection .
Opata, M. M. et al. B lymphocytes are required during the early priming of CD4 + T cells for clearance of pneumocystis infection in mice. J. Immunol. 195 , 611–620 (2015).
Rapaka, R. R. et al. Conserved natural IgM antibodies mediate innate and adaptive immunity against the opportunistic fungus Pneumocystis murina. J. Exp. Med. 207 , 2907–2919 (2010).
Steele, C. et al. Alveolar macrophage-mediated killing of Pneumocystis carinii f. sp. muris involves molecular recognition by the dectin-1 beta-glucan receptor. J. Exp. Med. 198 , 1677–1688 (2003).
Eddens, T. et al. Eosinophils contribute to early clearance of Pneumocystis murina infection. J. Immunol. 195 , 185–193 (2015).
Limper, A. H., Hoyte, J. S. & Standing, J. E. The role of alveolar macrophages in Pneumocystis carinii degradation and clearance from the lung. J. Clin. Invest. 99 , 2110–2117 (1997).
Li, X. V., Leonardi, I. & Iliev, I. D. Gut mycobiota in immunity and inflammatory disease. Immunity 50 , 1365–1379 (2019).
Underhill, D. M. & Braun, J. Fungal microbiome in inflammatory bowel disease: a critical assessment. J. Clin. Invest. 132 , e155786 (2022).
van Tilburg Bernardes, E. et al. Intestinal fungi are causally implicated in microbiome assembly and immune development in mice. Nat. Commun. 11 , 2577 (2020).
Rao, C. et al. Multi-kingdom ecological drivers of microbiota assembly in preterm infants. Nature 591 , 633–638 (2021).
Santus, W., Devlin, J. R. & Behnsen, J. Crossing kingdoms: how the mycobiota and fungal-bacterial interactions impact host health and disease. Infect. Immun. 89 , e00648-20 (2021).
Boutin, R. C. et al. Bacterial-fungal interactions in the neonatal gut influence asthma outcomes later in life. Elife 10 , e67740 (2021).
Bacher, P. et al. Human anti-fungal Th17 immunity and pathology rely on cross-reactivity against Candida albicans. Cell 176 , 1340–1355 e1315 (2019). This study shows that C. albicans -induced T H 17 cells cross-react with other fungi and can instigate pathology during Aspergillus -induced allergic lung disease .
Findley, K. et al. Topographic diversity of fungal and bacterial communities in human skin. Nature 498 , 367–370 (2013).
Sparber, F. et al. The skin commensal yeast malassezia triggers a type 17 response that coordinates anti-fungal immunity and exacerbates skin inflammation. Cell Host Microbe 25 , 389–403 e386 (2019). This study describes how type 17 immune responses in the skin can mediate protection against the commensal yeast Malassezia but can also exacerbate inflammation in injured skin .
Hurabielle, C. et al. Immunity to commensal skin fungi promotes psoriasiform skin inflammation. Proc. Natl Acad. Sci. USA 117 , 16465–16474 (2020).
Ost, K. S. et al. Adaptive immunity induces mutualism between commensal eukaryotes. Nature 596 , 114–118 (2021). This study uncovers the role of intestinal IgA responses in curtailing the pathogenic hyphal morphotype of C. albicans locally .
Doron, I. et al. Mycobiota-induced IgA antibodies regulate fungal commensalism in the gut and are dysregulated in Crohn’s disease. Nat. Microbiol. 6 , 1493–1504 (2021).
Doron, I. et al. Human gut mycobiota tune immunity via CARD9-dependent induction of anti-fungal IgG antibodies. Cell 184 , 1017–1031 e1014 (2021). This work shows that fungal colonization of the gut induces the generation of IgG responses that are dependent on CARD9 + CX3CR1 + mononuclear phagocytes and that protect from disseminated candidiasis .
Zhai, B. et al. High-resolution mycobiota analysis reveals dynamic intestinal translocation preceding invasive candidiasis. Nat. Med. 26 , 59–64 (2020). This human study shows that invasive candidiasis in recipients of HSCT is preceded by intestinal expansion and domination by pathogenic Candida .
Rolling, T. et al. Haematopoietic cell transplantation outcomes are linked to intestinal mycobiota dynamics and an expansion of Candida parapsilosis complex species. Nat. Microbiol. 6 , 1505–1515 (2021).
Malard, F. et al. Impact of gut fungal and bacterial communities on the outcome of allogeneic hematopoietic cell transplantation. Mucosal Immunol. 14 , 1127–1132 (2021).
Sokol, H. et al. Fungal microbiota dysbiosis in IBD. Gut 66 , 1039–1048 (2017).
Leonardi, I. et al. CX3CR1 + mononuclear phagocytes control immunity to intestinal fungi. Science 359 , 232–236 (2018).
Limon, J. J. et al. Malassezia is associated with Crohn’s disease and exacerbates colitis in mouse models. Cell Host Microbe 25 , 377–388 e376 (2019).
Jain, U. et al. Debaryomyces is enriched in Crohn’s disease intestinal tissue and impairs healing in mice. Science 371 , 1154–1159 (2021).
Iliev, I. D. et al. Interactions between commensal fungi and the C-type lectin receptor dectin-1 influence colitis. Science 336 , 1314–1317 (2012).
McGovern, D. P. et al. Genome-wide association identifies multiple ulcerative colitis susceptibility loci. Nat. Genet. 42 , 332–337 (2010).
Leonardi, I. et al. Fungal trans-kingdom dynamics linked to responsiveness to fecal microbiota transplantation (FMT) therapy in ulcerative colitis. Cell Host Microbe 27 , 823–829 e823 (2020).
Li, X. V. et al. Immune regulation by fungal strain diversity in inflammatory bowel disease. Nature 603 , 672–678 (2022).
Aykut, B. et al. The fungal mycobiome promotes pancreatic oncogenesis via activation of MBL. Nature 574 , 264–267 (2019).
Shiao, S. L. et al. Commensal bacteria and fungi differentially regulate tumor responses to radiation therapy. Cancer Cell 39 , 1202–1213 e1206 (2021).
Dohlman, A. B. et al. A pan-cancer mycobiome analysis reveals fungal involvement in gastrointestinal and lung tumors. Cell 185 , 3807–3822 e3812 (2022).
Narunsky-Haziza, L. et al. Pan-cancer analyses reveal cancer-type-specific fungal ecologies and bacteriome interactions. Cell 185 , 3789–3806 e3717 (2022). Dohlman et al. (2022) and Narunsky-Haziza et al. (2022) define the tumour-associated mycobiota across several human cancers and indicate that certain subtypes of cell-free or tumour-associated mycobiota may be useful as potential diagnostic or prognostic biomarkers in patients .
Lasbury, M. E., Tang, X., Durant, P. J. & Lee, C. H. Effect of transcription factor GATA-2 on phagocytic activity of alveolar macrophages from Pneumocystis carinii-infected hosts. Infect. Immun. 71 , 4943–4952 (2003).
Proctor, D. M. et al. Integrated genomic, epidemiologic investigation of Candida auris skin colonization in a skilled nursing facility. Nat. Med. 27 , 1401–1409 (2021).
Mathur, P. et al. Five-year profile of candidaemia at an Indian trauma centre: high rates of Candida auris blood stream infections. Mycoses 61 , 674–680 (2018).
CDC. Antibiotic resistance threats in the United States, 2019. https://www.cdc.gov/drugresistance/pdf/threats-report/2019-ar-threats-report-508.pdf (2019).
Eix, E. F. et al. Ex vivo human and porcine skin effectively model Candida auris colonization, differentiating robust and poor fungal colonizers. J. Infect. Dis. 225 , 1791–1795 (2022).
Huang, X. et al. Murine model of colonization with fungal pathogen Candida auris to explore skin tropism, host risk factors and therapeutic strategies. Cell Host Microbe 29 , 210–221.e6 (2020).
Bruno, M. et al. Transcriptional and functional insights into the host immune response against the emerging fungal pathogen Candida auris. Nat. Microbiol. 5 , 1516–1531 (2020).
Johnson, C. J., Davis, J. M., Huttenlocher, A., Kernien, J. F. & Nett, J. E. Emerging fungal pathogen Candida auris evades neutrophil attack. mBio 9 , e01403-18 (2018).
Oliveira, L. V. N., Wang, R., Specht, C. A. & Levitz, S. M. Vaccines for human fungal diseases: close but still a long way to go. NPJ Vaccines 6 , 33 (2021).
Rivera, A., Lodge, J. & Xue, C. Harnessing the immune response to fungal pathogens for vaccine development. Annu. Rev. Microbiol. 76 , 703–726 (2022).
Pappagianis, D., Valley Fever Vaccine Study Group. Evaluation of the protective efficacy of the killed Coccidioides immitis spherule vaccine in humans. Am. Rev. Respir. Dis. 148 , 656–660 (1993).
De Bernardis, F., Graziani, S., Tirelli, F. & Antonopoulou, S. Candida vaginitis: virulence, host response and vaccine prospects. Med. Mycol. 56 , 26–31 (2018).
Lin, L. et al. Th1-Th17 cells mediate protective adaptive immunity against Staphylococcus aureus and Candida albicans infection in mice. PLoS Pathog. 5 , e1000703 (2009).
Singh, S. et al. The NDV-3A vaccine protects mice from multidrug resistant Candida auris infection. PLoS Pathog. 15 , e1007460 (2019).
Spellberg, B. J. et al. Efficacy of the anti-Candida rAls3p-N or rAls1p-N vaccines against disseminated and mucosal candidiasis. J. Infect. Dis. 194 , 256–260 (2006).
Schmidt, C. S. et al. NDV-3, a recombinant alum-adjuvanted vaccine for Candida and Staphylococcus aureus, is safe and immunogenic in healthy adults. Vaccine 30 , 7594–7600 (2012).
Wuthrich, M. et al. Calnexin induces expansion of antigen-specific CD4 + T cells that confer immunity to fungal ascomycetes via conserved epitopes. Cell Host Microbe 17 , 452–465 (2015). This study shows that vaccine delivery of the conserved Blastomyces chaperone calnexin confers protection across multiple related fungal species .
Fisher, M. C. et al. Emerging fungal threats to animal, plant and ecosystem health. Nature 484 , 186–194 (2012).
Nilsson, R. H. et al. Mycobiome diversity: high-throughput sequencing and identification of fungi. Nat. Rev. Microbiol. 17 , 95–109 (2019).
Nash, A. K. et al. The gut mycobiome of the human microbiome project healthy cohort. Microbiome 5 , 153 (2017).
Auchtung, T. A. et al. Investigating colonization of the healthy adult gastrointestinal tract by fungi. mSphere 3 , e00092-18 (2018).
Download references
Acknowledgements
The authors apologize to their many colleagues whose valuable contributions they were not able to cite because of space constraints. This work was supported by the Division of Intramural Research of the NIAID, NIH (ZIA AI001175 to M.S.L.). R.A.D. was supported by the UK Medical Research Council (awards MR/S024611 and MR/T029137) and Academy of Medical Sciences (award SBF004_1008). T.M.H. was supported by NIH grants R01 AI093808, R01 AI139632, R21 AI156157 and P30 CA008748 (to Memorial Sloan Kettering Cancer Center).
Author information
Authors and affiliations.
Fungal Pathogenesis Section, Laboratory of Clinical Immunology, National Institute of Allergy and Infectious Diseases, National Institutes of Health, Bethesda, MD, USA
Michail S. Lionakis
Institute of Immunology and Immunotherapy, University of Birmingham, Birmingham, UK
Rebecca A. Drummond
Institute of Microbiology and Infection, University of Birmingham, Birmingham, UK
Infectious Disease Service, Department of Medicine, Memorial Sloan Kettering Cancer Center, New York, NY, USA
Tobias M. Hohl
Immunology Program, Sloan Kettering Institute, Memorial Sloan Kettering Cancer Center, New York, NY, USA
Human Oncology and Pathogenesis Program, Memorial Sloan Kettering Cancer Center, New York, NY, USA
Department of Medicine, Weill Cornell Medical College, New York, NY, USA
You can also search for this author in PubMed Google Scholar
Contributions
The authors contributed equally to all aspects of the article.
Corresponding author
Correspondence to Michail S. Lionakis .
Ethics declarations
Competing interests.
T.M.H. participated in a scientific advisory board for Boehringer Ingelheim in 2020. The other authors declare no competing interests.
Peer review
Peer review information.
Nature Reviews Immunology thanks A. Ibrahim, S. Levitz and the other, anonymous, reviewer(s) for their contribution to the peer review of this work.
Additional information
Publisher’s note Springer Nature remains neutral with regard to jurisdictional claims in published maps and institutional affiliations.
Supplementary information
(Als3). A Candida albicans hyphal protein that facilitates adhesion and invasion in host epithelial and endothelial cells through binding to E-cadherin and N-cadherin, respectively, and that has formed the basis for developing the investigational vaccine NDV-3.
A pore-forming, cytolytic peptide toxin produced by Candida albicans hyphae that promotes damage to oral epithelial cells and is required for virulence during mucosal candidiasis.
(CMC). A clinical condition characterized by severe, recurrent and treatment-refractory episodes of candidiasis of mucosal surfaces, skin and/or nails.
A life-threatening complication of uncontrolled diabetes that is characterized by insulin deficiency driving excessive accumulation of ketone bodies in the blood, which leads to acidosis.
Long filamentous branching structures of certain fungi (for example, Aspergillus ) that grow by apical extension.
Conditions caused by deleterious germ line mutations in single immune-related genes that manifest themselves with various infectious complications with or without other accompanying clinical manifestations, such as autoimmunity, autoinflammation, atopy and/or malignancy.
A family of serine proteases (kallikreins) that cleave kininogens to generate the kinin peptides bradykinin and kallidin, which as a collective system have important roles in renal homeostasis and blood pressure regulation, with additional roles now emerging during inflammation, immunity and tissue repair.
A non-canonical autophagy pathway activated downstream of pattern recognition receptors which promotes phagosome maturation and intracellular killing of pathogens within phagocytes and regulates other mononuclear phagocyte functions, such as antigen presentation, efferocytosis and anti-inflammatory cytokine responses.
A type 17 cell subpopulation that develops in the thymus, expresses CD4, TCRαβ, RORγt, CCR6 and IL-23R and, in contrast to inducible T H 17 cells, is characterized by an intrinsic capacity for rapid activation in response to T cell receptor-independent stimulation in naive hosts.
The ability of the innate immune system, through epigenetic and metabolic reprogramming, to develop a form of immunological memory that can provide protection against a subsequent challenge with homologous or even heterologous pathogens.
A clinical syndrome characterized by increased concentrations of urea in the blood and electrolyte, volume and metabolic abnormalities that are collectively caused by deteriorating kidney function.
A eukaryotic, unicellular fungal organism that reproduces by budding (for example, Candida ).
Rights and permissions
Reprints and permissions
About this article
Cite this article.
Lionakis, M.S., Drummond, R.A. & Hohl, T.M. Immune responses to human fungal pathogens and therapeutic prospects. Nat Rev Immunol 23 , 433–452 (2023). https://doi.org/10.1038/s41577-022-00826-w
Download citation
Accepted : 01 December 2022
Published : 04 January 2023
Issue Date : July 2023
DOI : https://doi.org/10.1038/s41577-022-00826-w
Share this article
Anyone you share the following link with will be able to read this content:
Sorry, a shareable link is not currently available for this article.
Provided by the Springer Nature SharedIt content-sharing initiative
This article is cited by
Comparison of metagenomic next-generation sequencing and conventional culture for the diagnostic performance in febrile patients with suspected infections.
BMC Infectious Diseases (2024)
Activation of leukotriene B4 receptor 1 is a prerequisite for complement receptor 3-mediated antifungal responses of neutrophils
- Sihan Xiong
Cellular & Molecular Immunology (2024)
Immunoinformatic-guided designing and evaluating protein and mRNA-based vaccines against Cryptococcus neoformans for immunocompromised patients
- Amir Elalouf
- Amit Yaniv-Rosenfeld
Journal of Genetic Engineering and Biotechnology (2023)
Newly emerging type B insulin resistance (TBIR) during treatment with eculizumab for AQP4-IgG-positive neuromyelitis optica spectrum disorder (NMOSD): fatal outcome
- S. Doubrovinskaja
- M. Korporal-Kuhnke
- B. Wildemann
Journal of Neurology (2023)
Quick links
- Explore articles by subject
- Guide to authors
- Editorial policies
Sign up for the Nature Briefing newsletter — what matters in science, free to your inbox daily.

share this!
April 8, 2024
This article has been reviewed according to Science X's editorial process and policies . Editors have highlighted the following attributes while ensuring the content's credibility:
fact-checked
peer-reviewed publication
trusted source
An inside look at how plants and mycorrhizal fungi cooperate
by Lawrence Berkeley National Laboratory
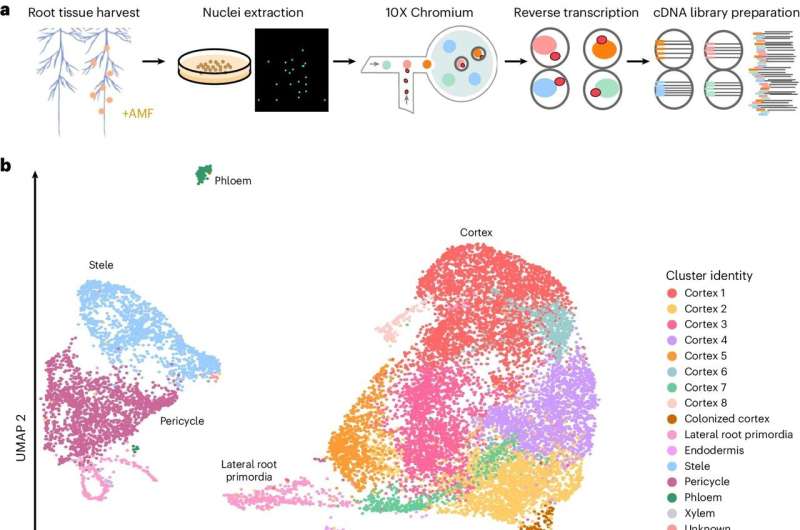
For millions of years, underground fungi have lived in symbiosis with plant roots. Plants provide photosynthesized carbon, while fungi deliver water and nutrients. In order to do so, these organisms share space at the cellular scale: fungi stretch a network of tendrils called arbuscules into a plant's root cells, and both organisms rearrange their cells around this structure to facilitate sharing.
Recently, researchers have been able to study both sides of this interaction up close, using RNA sequencing to understand gene expression: one of the first cross-kingdom spatially-resolved transcriptomics studies to date. This paper appears as a cover article in Nature Plants .
"We wanted to better understand the nature of this symbiosis at the cellular level—really understand how those two cell types [of two different organisms] are interacting with each other, without all the noise or other biological activity of the surrounding neighborhood," said Benjamin Cole, senior author of this work.
Cole is a research scientist at the U.S. Department of Energy (DOE) Joint Genome Institute (JGI), a DOE Office of Science User Facility located at Lawrence Berkeley National Laboratory (Berkeley Lab).
A specific understanding of this symbiosis could offer improvements in multiple directions. On the fungi side, underground mycorrhizal networks can serve as a reservoir for carbon compounds that plants generate from the carbon dioxide they take in. In this way, encouraging this symbiosis could improve the way soils store carbon from the atmosphere. For plants, boosting this relationship could improve biofuel feedstocks growing in nutrient-poor fields.
"This is one interaction that allows plants to better survive in those environments," Karen Serrano said. She is the first author of this paper and a graduate researcher at the Joint BioEnergy Institute (JBEI).
Cole led the work in collaboration with JBEI as part of the 2021 Laboratory Directed Research and Development (LDRD) program. Cole also received a DOE Early Career Research Program (ECRP) award in 2021, which aims in part to build off of this work. Former JGI postdoctoral scholar Margot Bezrutczyk collaborated extensively with Cole and Serrano to collect and analyze the single nuclei and spatial transcriptomics data this work leverages.
These experiments focused on two species at once: the model legume species Medicago truncatula and the mycorrhizal fungi Rhizophagus irregularis.
To see how these organisms cooperate, this team applied R. irregularis spores directly to M. truncatula seedlings grown in a controlled environment chamber so the fungus could colonize the plants' roots. Then, comparing with control seedlings which were not treated with fungi, they used multiple approaches to look at gene expression in both plant cells and fungal cells.
Using a technique called single nuclei RNA sequencing, the researchers identified different cell types within M. truncatula root cells and profiled their gene expression.
Then, the researchers used a technique called spatial transcriptomics to generate maps of gene expression. This spatial transcriptomics technique allowed them to understand gene expression within circular capture areas roughly 55 microns in diameter—about the width of a human hair. At such resolution, this spatial transcriptomics data captured molecular information from both plant cells and fungal cells.
"Because this technology relies on just polyadenylated transcript capture—any RNA that is from eukaryotes—we were able to capture both plant and fungal transcripts," Serrano said. This team quantified the expression of over 12,000 fungal genes, in addition to associated plant genes.
Altogether, these data offer a granular view of both plant and fungal activity at different stages of this symbiosis. Within that activity, Serrano, Cole, and their team found over 1,000 upregulated genes, 188 of which were shared with previous studies in the same system. With the right functional characterization, those genes could become dials for tuning this symbiosis. "Those are great candidates for genetic engineering. Our hope is that the community at large will follow that up," Serrano said.
This work focused on a relatively well-understood model system, so future directions will also include targeting biofuel feedstocks in similar studies. "We would like to look at arbuscular mycorrhizal symbiosis in other bioenergy grasses, like sorghum and switchgrass. We're optimizing systems now so that we can get those working," Cole said.
Journal information: Nature Plants
Provided by Lawrence Berkeley National Laboratory
Explore further
Feedback to editors

Researchers find baby stars discharge plume-like 'sneezes' of magnetic flux during formation
5 minutes ago

Oxidant pollutant ozone removes mating barriers between fly species, study finds

Nothing is everything: How hidden emptiness can define the usefulness of filtration materials

Cloud engineering could be more effective 'painkiller' for global warming than previously thought

Pork labeling schemes 'not helpful' in making informed buying choices, say researchers

Ocean currents threaten to collapse Antarctic ice shelves, study finds

Using CO₂ and biomass, researchers find path to more environmentally friendly recyclable plastics
12 hours ago

Precision agriculture research identifies gene that controls production of flowers and fruits in pea plants

Long-term forest study shows tornado's effects linger 25 years later

A new coating method in mRNA engineering points the way to advanced therapies
Relevant physicsforums posts, how to implement beamforming in ultrasound diffraction tomography.
16 hours ago
Potentially fatal dog parasite found in the Colorado River
Apr 9, 2024
What do large moles on the body indicate?
Mar 30, 2024
Avian flu - A new study led by a team from the University of Maryland
Mar 27, 2024
Are all biological catabolic reactions exergonic?
Mar 20, 2024
A First of Its Kind: A Calcium-based signal in the Human Brain
Mar 18, 2024
More from Biology and Medical
Related Stories

A plant root atlas for tracking developmental trajectories
Mar 8, 2022

Discovery of the interactions between plants and arbuscular mycorrhizal fungi
Aug 11, 2022

Exploring the genetic composition of fungi and its role in plant health
Mar 7, 2024

The underground network: Decoding the dynamics of plant-fungal symbiosis
Jan 25, 2024

Alternative to phosphate fertilizer: Biochar basis controls plant response
Aug 2, 2023

The enemy within: How a killer hijacked one of nature's oldest relationships
Feb 28, 2020
Recommended for you

Ants in Colorado are on the move due to climate change
14 hours ago

Smart vest turns fish into underwater spies, providing a glimpse into aquatic life like never before

Caterpillar 'noses' are surprisingly sophisticated, researchers find

Single-crop farming has potential to harm bees, study finds
17 hours ago
Let us know if there is a problem with our content
Use this form if you have come across a typo, inaccuracy or would like to send an edit request for the content on this page. For general inquiries, please use our contact form . For general feedback, use the public comments section below (please adhere to guidelines ).
Please select the most appropriate category to facilitate processing of your request
Thank you for taking time to provide your feedback to the editors.
Your feedback is important to us. However, we do not guarantee individual replies due to the high volume of messages.
E-mail the story
Your email address is used only to let the recipient know who sent the email. Neither your address nor the recipient's address will be used for any other purpose. The information you enter will appear in your e-mail message and is not retained by Phys.org in any form.
Newsletter sign up
Get weekly and/or daily updates delivered to your inbox. You can unsubscribe at any time and we'll never share your details to third parties.
More information Privacy policy
Donate and enjoy an ad-free experience
We keep our content available to everyone. Consider supporting Science X's mission by getting a premium account.
E-mail newsletter
Language selection
- Français fr
Call for papers: Generating stronger evidence to inform policy and practice: natural experiments on built environments, health behaviours and chronic diseases

HPCDP Journal Home

Submit a manuscript
- Information for authors
About HPCDP
- About the Journal
- Contact the Editors
- Past issues
Previous | Table of Contents | Next
https://doi.org/10.24095/hpcdp.44.4.05

Recommended Attribution
This call for papers in the HPCDP Journal is licensed under a Creative Commons Attribution 4.0 International License
Guest editors: Dr. Stephanie Prince Ware (Public Health Agency of Canada), Dr. Gavin McCormack (University of Calgary)
HPCDP Journal Editors: Robert Geneau and Margaret de Groh (Public Health Agency of Canada)
Where we work, learn, play, eat and live has important implications for health. The built environment has been associated with the development of chronic disease, and with health behaviours often seen as critical pathways for this relationship. Footnote 1 Footnote 2 Built environments refer to components of the physical environment that are human-made or human-modified and include structures and buildings, recreation facilities, green spaces and parks, transportation systems and community design.
Natural experiments are interventions that occur without a researcher’s ability to manipulate the intervention or exposure to the intervention. Footnote 3 Footnote 4 Natural experiments offer the opportunity to evaluate the effects of “naturally occurring” interventions such as changes to the built environment (e.g. creation of a new bike path, park improvements, infrastructure changes to schools or workplaces, construction of a new recreation facility or grocery store) on health behaviours and chronic disease risk. Natural experiments are often more practical for investigating the health impacts of environmental interventions when compared to traditional experimental studies (e.g. randomized controlled trials). Compared to cross-sectional studies, natural experiments provide a means to generate rigorous evidence to better establish causality, as well as to understand the implementation of interventions in “real-world” scenarios.
This special issue answers the 2017 Canadian Public Health Officer annual report’s call to further evaluate the health impacts of community design features in Canada. Footnote 5 This special issue resonates with the expanding scholarly and policy-oriented interest in the utility of natural experiments as a critical tool in advancing the body of evidence and for informing interventions to improve public and population health. Footnote 6 Footnote 7 Specifically, the objective of this special issue on natural experiments is to provide timely evidence to further understand the effectiveness of built environment interventions on health behaviours and chronic disease prevention in a Canadian context.
Health Promotion and Chronic Disease Prevention in Canada: Research, Policy and Practice is seeking relevant topical research articles that present new findings or synthesize/review existing evidence on natural experiments of the built environment (or related policies) that influence health behaviours with implications for chronic disease prevention in Canada.
Relevant topic areas include, but are not limited to:
- Built environments, including community or neighbourhoods, workplaces, schools, transportation infrastructure, home environments, recreation environments, parks, playgrounds, green spaces, public open spaces, natural environments and seniors’ residences.
- All health-related behaviours, including physical activity, sedentary behaviour, sleep, food consumption, smoking and substance use.
- Chronic diseases and health-related outcomes, including body mass index, fitness, blood pressure, blood lipids, blood sugar, injuries, falls, mental health, stress, depression, anxiety, Alzheimer's disease, dementia, obesity, metabolic syndrome, cardiovascular disease, cancer, diabetes and lung disease.
International submissions will be considered if they include Canadian data, results (e.g. as part of multi-country studies or global comparisons) and/or evidence-based discussion of implications for community or population health in Canada.
Consult the Journal’s website for information on article types and detailed submission guidelines for authors . Kindly refer to this call for papers in your cover letter.
All manuscripts should be submitted using the Journal’s ScholarOne Manuscripts online system. Pre-submission inquiries and questions about suitability or scope can be directed to [email protected] .
Submission deadline: November 30, 2024
Page details
Impact of research on maize production challenges in Hungary
- Ssemugenze, Brian
- Ocwa, Akasairi
- Bojtor, Csaba
- Illés, Árpád
- Esimu, Joseph
- Nagy, János
Maize (Zea mays L), as a major cereal crop produced in Hungary in addition to wheat, attracts enormous research from both educational and non-educational institutions. Research is aimed at addressing the key abiotic, biotic and social economic constraints. The stakeholders and institutions involved in research are spread all over Hungary. Currently, no review has been done to comprehensively reveal the trend of maize research in Hungary, as well as key players such as institutions, universities, industry and researchers. Hence, this bibliographic review was conducted to: i) identify the major research institutions and their contribution towards maize research in Hungary; ii) evaluate the major maize research areas in Hungary between 1975 and 2022. Literature search was conducted in Web of Science (WoS) database using keywords; 'maize' OR 'maize' + 'Research' + 'Hungary'. Bibliometric analyses were performed using the VOSviewer software. Changes in the publication trend of documents was tested using Mann Kendall Test. A total of 947 publications related to the topic were published by 441 institutions between 1975 and 2022. There was a significant (p = 0.001) positive increase in the number of published documents. Hungarian Academy of Science (210 documents) and University of Debrecen (132 documents) recorded the highest number of publications contributing 58.7% of the maize research literature in Hungary. The major research areas included: increasing maize yield, hybrid development, pests and diseases, irrigation, fertilization (nitrogen), drought, temperature, gene expression and climate change. The increasing number of published documents signifies an improved response to addressing maize production challenges through research in order to boost its productivity.

An official website of the United States government
The .gov means it’s official. Federal government websites often end in .gov or .mil. Before sharing sensitive information, make sure you’re on a federal government site.
The site is secure. The https:// ensures that you are connecting to the official website and that any information you provide is encrypted and transmitted securely.
- Publications
- Account settings
Preview improvements coming to the PMC website in October 2024. Learn More or Try it out now .
- Advanced Search
- Journal List

Recent advances in therapies for onychomycosis and its management
Aditya k. gupta.
1 Division of Dermatology, Department of Medicine, University of Toronto School of Medicine, Toronto, Canada
2 Mediprobe Research Inc., London, Ontario, Canada
Onychomycosis is the most common affliction of the nail. It may be caused by dermatophytes, yeasts, and non-dermatophyte molds. Traditionally, oral antifungal treatments have been used to treat the fungus, although they can be accompanied by side effects and drug interactions. Topical treatments provide an alternative modality, bypassing the systemic effects of oral drugs; recent research has centered on topical drug improvement and development. Physical and laser treatments are being used in conjunction with topicals, which may help penetrate the thick nail plate. In this review, techniques from all categories are outlined: both novel experimental approaches and progress and effectiveness of recently developed treatments. More long-term studies are required to determine the efficacy of various treatments, but cure rates are improved when patients adhere to treatments and follow preventative measures to avoid disease recurrence.
Introduction
Onychomycosis is a fungal infection occurring in the nails and may affect the adjacent skin. Typically, it manifests as discoloration of the nail, nail plate thickening, and onycholysis 1 . It is the most common nail pathology and accounts for about 90% of toenail infections worldwide 1 . This infection presents several problems for affected populations, including local pain, paresthesia, and reduced quality of life as its appearance may impair social interactions and daily activities 2 . Most onychomycoses are caused by the dermatophytes Trichophyton , Trichophyton rubrum , Trichophyton mentagrophytes , and Epidermophyton 3 . Infections often initiate from tinea pedis, a fungal infection on the surrounding skin of the feet 3 . Factors that contribute to disease progression are humidity, occlusive footwear, nail trauma, and genetic predisposition 4 , 5 . Patients with diabetes, poor peripheral circulation, HIV, and immunosuppression are also more susceptible, as are elderly patients 6 – 10 .
Owing to its chemical composition, the nail is a formidable barrier to the permeation of drugs, and diffusion into the nail is poor relative to the skin 11 . This, coupled with slow toenail growth, requires that topicals be used for 12 months or longer, ideally until a healthy nail has regrown. Traditionally, oral therapeutics have been the preferred treatment because of their accessibility and efficacy 2 . Terbinafine and itraconazole are US Food and Drug Administration (FDA)–approved oral antifungal medications, and fluconazole is available as an off-label option in the US. Though effective, they may be accompanied by systemic side effects and drug–drug interactions, which is a concern for those already taking medications for other conditions. Patients may be hesitant to take oral antifungals. It is understandable that there is demand for non-systemic treatments. At present, there are several oral, topical, and physical therapies broadening the treatment options available to patients. Additionally, combination therapy with several drug classes/modalities can be considered 12 . Some research groups are studying new molecules and permeation enhancers for topicals, diversifying drug targets, and improving the effectiveness of base molecules already in use. When the efficacy of these drugs is measured, commonly used outcome measures are mycological cure and complete cure. Mycological cure is defined as eradication of the fungal pathogen from the nail, confirmed by a negative potassium hydroxide (KOH) preparation (a test to differentiate dermatophytes and yeasts from other skin disorders) and negative fungal culture. Complete cure meets the goals of the patient, physician, and regulatory bodies. Complete cure is defined as 100% clear nail (also known as clinical cure) in addition to mycological cure 13 . This review will outline some of these recent developments in therapies for managing onychomycosis.
Building on topical therapies
Topical therapies can effectively treat onychomycosis, particularly when patients adhere to treatment instructions. Owing to the structural nature of the nail, research is focused on improving the delivery of the drug transungually to the nail bed where fungus lies. Unlike their oral counterparts, topical treatments are relatively safe and there is no potential for drug–drug interactions. Properties that influence the permeability of the drug through the nail include molecular weight, lipophilicity, affinity to keratin, ionization, pH, and the ability to sublimate 14 . It is advantageous to develop a drug delivery system that allows the drug to enter the nail plate through the transungual and subungual routes rather than just penetrating the nail plate, which is demonstrated by the application of tavaborole and efinaconazole 15 . Phase III clinical trials have been completed for these drugs and further studies are in progress to understand long-term efficacy 14 . Despite significant advances in the effectiveness of topical treatments, mycological and complete cure rates remain relatively lower than those of some of the oral agents ( Table 1 ). However, clinical response rates (resulting in cosmetically clear nails) are more favorable.
Tavaborole is a novel boron-based antifungal agent that was approved by the FDA for treating onychomycosis in 2014 16 . It penetrates the nail plate because of its small molecular weight and interferes with protein synthesis in fungal cells through its effects on cytoplasmic aminoacyl-tRNA synthetases 17 . Phase II and III trials have demonstrated its safety and effectiveness for treating mild to moderate onychomycosis (20 to 60% nail involvement). In two phase III randomized, double-blind, vehicle-controlled trials, tavaborole 5% solution was applied once daily for 48 weeks, and efficacy was evaluated at 52 weeks. Patients ranged from 18 to 88 years of age. Mycological cure rates (negative KOH and culture) with tavaborole 5% solution for studies 1 and 2 were 31.1% and 35.9%, respectively, significantly higher than vehicle (7.2% and 12.2%, P <0.001). Complete cure rates (100% clear nail and mycological cure) were significantly higher for tavaborole compared with vehicle in study 1 (6.5% and 0.5%, P = 0.001) and study 2 (9.1% and 1.5%, P <0.001) 18 . Similarly, a pooled post-study follow-up from these two trials showed that complete cure was higher in the tavaborole-treated group compared with the vehicle control group (28.6% versus 7.7%) at week 60. Additionally, at 60 weeks, mycological cure for the tavaborole group was higher than vehicle (53.1% versus 23.1%) 19 .
Efinaconazole 10% solution was FDA-approved as a treatment around the same time in 2014. It is a triazole antifungal that inhibits the synthesis of ergosterol in the fungal cell wall. In phase III trials, patients with distal lateral subungual onychomycosis (20 to 50% nail involvement, 18 to 71 years of age) received the solution once daily for 48 weeks and were evaluated at 52 weeks. The results were a 17.8% complete cure rate (0% clinical involvement of nail, negative KOH and culture) versus 3.3% for vehicle in study 1 and 15.2% versus 5.5% in study 2 ( P <0.001). Mycological cure rates (negative KOH and culture) were significantly higher with efinaconazole (55.2% in study 1 and 53.4% in study 2) compared with vehicle (16.8% and 16.9%, P <0.001) 12 , 22 .
Luliconazole was approved in 2013 for fungal infections of the skin, including tinea pedis in the US 25 . A 10% cream was investigated as a treatment for onychomycosis. In separate phase IIb/III clinical trials, nail samples were isolated from patients to compare the activity of 10% luliconazole with amorolfine, ciclopirox, and terbinafine against distal subungual onychomycosis 26 . It showed a mean minimum inhibitory concentration of 0.00022 μg/mL, which was lower than that of the other three antifungals. In a Japanese multicenter, double-blind, randomized phase III study, patients 21 to 79 years of age with 20 to 50% nail involvement were placed into 2:1 groups of once-daily application of luliconazole 5% nail solution and vehicle. After 48 weeks, complete cure (0% clinical involvement of the nail and negative direct microscopy) rate was significantly higher in luliconazole groups (14.9%) compared with vehicle (5.1%, P = 0.012). Similarly, the negative direct microscopy rate was significantly higher in luliconazole (45.4%) than vehicle (31.2%, P = 0.026). It is suggested that once-daily topical application of luliconazole is clinically effective and well tolerated 27 . Luliconazole is not approved for the treatment of onychomycosis in the US.
In Europe, ciclopirox 8% hydrolacquer (P-3051) uses a novel technology based on hydroxypropyl chitosan for the delivery of ciclopirox 8% to the nail 28 . In a randomized, evaluator-blinded, controlled, parallel-group clinical trial, P-3051 showed statistical superiority to amorolfine after 48 weeks in complete cure (negative KOH and culture and no residual clinical involvement of the nail, 35% versus 11.7%, respectively, P <0.001) in 120 patients 18 to 75 years of age with 25 to 75% nail involvement. Similarly, mycological cure (negative direct microscopy and culture) was achieved by all patients who received P-3051 compared with 81.7% who received amorolfine ( P <0.001) 29 . In a randomized, evaluator-blinded, placebo-controlled, parallel-group clinical trial comparing P-3051 with reference ciclopirox 8% and placebo, 467 patients (mean age of 49.84 ± 11.89 years) with 25 to 60% nail involvement applied the lacquers for 48 weeks, followed by a 4-week washout period and 8-week follow-up period 28 . Complete cure (negative KOH microscopy, culture, and 100% growth of a healthy nail at week 48 and washout) was achieved in 5.7% of P-3051 users and 3.2% for reference ( P = 0.6834), whereas placebo saw no cure ( P = 0.0165). P-3051 complete cure rate increased at 60 weeks (12.7%) and was greater than reference (5.8%, P <0.05) and placebo (1.3%, P = 0.0029). A post-hoc analysis confirmed that severity of disease is a prognostic factor for responsiveness to P-3051 treatment and significantly affects reported efficacy data 30 . The population subset excluded patients with more severe disease (>50% nail involvement). P-3051 was superior to placebo and reference ciclopirox in cure and response rates at 60 weeks, and efficacy rates in the P-3051 group were higher in the groups that excluded patients with more than 50% nail involvement. Ciclopirox 8% hydrolacquer (P-3051) is not approved for the treatment of onychomycosis in the US.
A study using polyurethanes (PUs) as new excipients in topical nail treatments has been conducted 31 . A PU polymer delivered two antifungal drugs (terbinafine and ciclopirox), and a 10% PU concentration was most effective for in vitro drug release, permeation, and antifungal activity. The lacquer smooths the nail plate and reduces porosity, increasing effectiveness of the base molecule 31 . Finally, there are ongoing clinical trials for ME-1111 and MOB-015. ME-1111 is a new agent with potent in vitro antifungal activity and small molecular weight 32 , 33 . It targets succinate dehydrogenase of the electron transport chain, inhibiting it and blocking ATP production. MOB-015 is a topical formulation of terbinafine 34 .
Oral therapies
Typically, oral therapeutics are reserved for severe infections because of their safety issues and drug–drug interactions 35 . Recently, there has been insight about the use of ravuconazole and its prodrugs as new drug candidates for oral therapy. A water-soluble prodrug, mono-lysine phosphoester derivative (BFE1224), is in the advanced stages of clinical development 35 . A phase III randomized, double-blind, placebo-controlled study of fosravuconazole (F-RVCZ) L-lysine ethanolate, the novel oral triazole, was conducted in Japan 36 . One hundred fifty-three patients 20 to 75 years of age with at least 25% nail involvement were assigned 100 mg of F-RVCZ or placebo to take once daily for 12 weeks. Evaluation was carried out at week 48. The complete cure rates (0% nail involvement and negative KOH) for F-RVCZ and placebo were 59.4% and 5.8%, respectively ( P <0.001). Mycological cure rate (negative KOH) was determined every 12 weeks and increased over time; the difference between F-RVCZ and placebo was statistically significant at 24 weeks and onward ( P = 0.002 and P <0.001 at weeks 36 and 48). At week 48, mycological cure rates were 82.0% for F-RVCZ and 20.0% for placebo 36 . Fosravuconazole (F-RVCZ) is not approved for the treatment of onychomycosis in the US.
VT-1161 is a novel, tetrazole fungal CYP51 inhibitor designed to selectively target fungal enzymes and maintains high potency for the fungal target 37 . In a randomized, phase 2b study to evaluate the efficacy and safety of oral VT-1161 for onychomycosis, 259 patients 18 to 70 years of age with 25 to 75% nail involvement at baseline received 300 or 600 mg oral doses or matching placebo. Once-weekly VT-1161 at a dose of 300 or 600 mg was administered for 10 or 22 weeks following a 14-day once-daily loading period at the same dose (300 or 600 mg) 38 . Mycological cure (negative KOH and culture) at week 48 was shown for 61%to 72% of patients, collapsed across VT-1161 arms, whereas complete cure ranged from 32 to 40% 38 . Phase II clinical trials have been completed 39 .
Physical strategies and laser therapies
Heinlin et al . 40 showed that repeated, daily cold atmospheric plasma treatment inhibits the in vitro growth of T. rubrum . Recently, a pilot study demonstrated the effects of non-thermal plasma in treating onychomycosis 41 . Non-thermal plasma was created by using an electric insulator by short pulses (10 ns) of electric fields that ionize air molecules. This process creates ions, electrons, ozone, hydroxyl radicals, and nitric oxide, which are fungicidal and cytotoxic 41 . Ultimately, 13 patients 33 to 74 years of age with 25 to 50% nail involvement completed the trial, and 15.4% achieved mycological cure (negative KOH and culture). Additional studies are required because of the small sample size and varying protocols, but it is the first clinical study to report that thermal plasma may be effective against toenail onychomycosis.
Another novel physical strategy is precise laser poration 42 . Hollow-core photonic crystal fibers guide light from a femtosecond-pulsed laser in a focused, high-energy density beam. As it irradiates the nail, the laser creates pores (100 μm in diameter) with minimal damage to surrounding tissues. Complete poration of nails increases permeation by two to three orders of magnitude relative to an untreated nail, which in turn would increase the effectiveness of topical treatments 42 . Flores et al . 43 coupled poration with a nanocapsule formulation of tioconazole. The nano-formulations are advantageous for topical delivery because they ensure stability of actives and act as reservoirs for extended drug delivery. Nanocapsules were composed of pullulan, a water-soluble polysaccharide that forms films 44 . The newer film-forming formula provided the best efficiency of ex vivo delivery, and drug payload percentages were higher than those of marketed products.
Lasers are approved by the FDA because of their similarity to predicate devices. These devices improve cosmetic appearance by increasing the clarity of nail in patients with onychomycosis 12 , 45 , 46 . The effectiveness of lasers as a standalone treatment is reported inconsistently, and cure rates for laser treatment are lower than those of oral and topical treatments. There is limited evidence that they can eradicate pathogenic fungi and this is due to incomplete reporting of randomization and lack of controls 47 – 49 . Additionally, the inclusion criteria and definitions of efficacy outcomes between drug and devices differ, preventing meaningful comparisons 46 . In the US, lasers are approved for the temporary increase of clear nail in onychomycosis 50 .
Commonly used lasers include neodymium-yttrium garnet lasers and Q-switched laser systems 1 . The smaller temporal pulse length in this laser is less than the thermal relaxation time of the fungi, which permits contained heating of the fungi in the nail plate while allowing dissipation of heat in the surrounding soft tissue of the toenail and fingernail. There is a lack of robust clinical data. Randomized, double-blind trials are required to determine whether they are actually fungicidal 51 , 52 .
Adjunctive measures
Aside from adhering to the prescription protocol of their onychomycosis therapy, patients can perform measures to improve the effectiveness of their treatment and avoid the possibility of reinfection. They should disinfect shoes and socks, avoid walking barefoot in public places, keep feet cool and dry, and recognize the early signs of recurrence and reinfection 4 , 53 . It is also important to treat tinea pedis, and any affected family members, early and effectively.
Conclusions
There is a diverse array of therapies for treating onychomycosis, particularly centered on topical formulas as the adverse effects are limited to the application site without systemic drug interactions. Devices are being considered as an addition to antifungal therapies, which is an important step in diversifying treatment options 1 .
Abbreviations
FDA, US Food and Drug Administration; KOH, potassium hydroxide; PU, polyurethane.
[version 1; peer review: 2 approved]
Funding Statement
The author(s) declared that no grants were involved in supporting this work.
Editorial Note on the Review Process
F1000 Faculty Reviews are commissioned from members of the prestigious F1000 Faculty and are edited as a service to readers. In order to make these reviews as comprehensive and accessible as possible, the referees provide input before publication and only the final, revised version is published. The referees who approved the final version are listed with their names and affiliations but without their reports on earlier versions (any comments will already have been addressed in the published version).
The referees who approved this article are:
- Evan Rieder , The Ronald O. Perelman Department of Dermatology, New York University School of Medicine, New York, NY, USA No competing interests were disclosed.
- Chris G. Adigun , Dermatology & Laser Center of Chapel Hill, Chapel Hill, NC, USA No competing interests were disclosed.
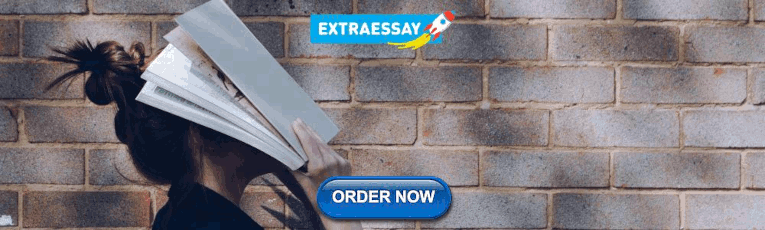
IMAGES
VIDEO
COMMENTS
Breaking the mould: rethinking 'wild type' in fungal pathogens. In this Journal Club, Amelia Barber discusses a study revealing intraspecies heterogeneity in a fungal pathogen, prompting us to ...
Feature papers represent the most advanced research with significant potential for high impact in the field. A Feature Paper should be a substantial original Article that involves several techniques or approaches, provides an outlook for future research directions and describes possible research applications. ... Journal of Fungi is an ...
Fungi are morphologically and ecologically diverse kingdom but less explored in the global perspective. This systematic review of mainly higher fungi (mushrooms) and lichenized fungi (lichens) was aimed to convey comprehensive knowledge on these understudied taxa, especially considering diversity, research trends, taxonomic/geographic knowledge gaps, and their contribution to ecosystem services.
The paper presents new localities of the fungus Clathrus archeri (Berk.) Dring (Phallaceae) in Greater Poland. New data from 2022 from the Pleszew area have been provided.
Introduction. Despite their perception as something found in the forest or served up on a dinner plate, fungi are more than just mushrooms. They span an impressive range of sizes, from microscopic cells to among the largest organisms on Earth (Sipos et al. 2018), and have a major impact on human health, agriculture, biodiversity, ecology, manufacturing, and biomedical research.
Fungi have been used to better the lives of everyday people and unravel the mysteries of higher eukaryotic organisms for decades. However, comparing progress and development stemming from fungal research to that of human, plant, and bacterial research, fungi remain largely understudied and underutilized.
Abstract. Invasive fungal infections pose an important threat to public health and are an under-recognized component of antimicrobial resistance, an emerging crisis worldwide. Across a period of ...
The potential of fungi for a more sustainable world must be released to address global challenges of climate change, higher demands on natural resources, and the increased burden of life-style diseases. Genome sequencing was developed first for the human genome after which bacterial genomes were quickly sequenced.
The Kingdom Fungi, home to molds, mushrooms, lichens, rusts, smuts. and yeasts, comprises eukaryotes. with remarkably diverse life histories. that make essential contributions. to the biosphere ...
Fungi have the ability to transform organic materials into a rich and diverse set of useful products and provide distinct opportunities for tackling the urgent challenges before all humans. Fungal biotechnology can advance the transition from our petroleum-based economy into a bio-based circular economy and has the ability to sustainably produce resilient sources of food, feed, chemicals ...
Fungi are recognized by neutrophils, macrophages, monocytes, ... The function of PRRs (especially CLR-related research), impaired IL-17 responses, aberrant type 1-associated T cell responses, the impact of cutaneous sensory neurons, and the presence of candidalysin ... Papers of particular interest, published within the period of review, have ...
PDF | On Jan 1, 2012, Lori M. Carris and others published Introduction to Fungi | Find, read and cite all the research you need on ResearchGate
The current review paper narrates the contribution of fungi in bio-economies. A greater understanding of the roles and value of fungi can be used to build global support for mycological appreciation. It will, in particular, raise policymakers' awareness of the importance of fungi, leading to future research and conservation efforts ...
Thus, interest in fungal immunology research has increased in recent years, catalysed by our growing understanding of the biology and functions of C-type lectin receptors (CLRs) 5,6, which are the ...
Therefore, fungal endophytes have been extensively studied for their beneficial properties and potential to enhance productivity for crop plants. The literature research conducted in this paper reveals that studies focused on PGPF take near 20% of the fungal endophyte investigation, covering both in vitro and in field application on plants.
In 2009, a ∼530 nm maximum wavelength of green light emission in fungi was confirmed to be the result of an enzyme-mediated reaction in which a hot extract containing heat-stable factors (substrate/luciferin) mixed with a cold extract containing enzymes (luciferase) [12].The same team went on to detect bioluminescence from combinations of substrates/enzymes in different fungal lineages ...
Recent research demonstrates that the number of virulent phytopathogenic fungi continually grows, which leads to significant economic losses worldwide. Various procedures are currently available for the rapid detection and control of phytopathogenic fungi. Since 1940, chemical and synthetic fungicides were typically used to control ...
Rising Stars in Fungal Pathogenesis: 2023. Brian Wickes. Yong-Sun Bahn. Michal Adam Olszewski. Adriana Marcela Celis Ramírez. Hokyoung Son. 1,043 views. 3 articles. An innovative journal that brings together different fields of mycology - agricultural, medical and applied industrial - to decipher the interactions of fungi, aiming to improve ...
Cole led the work in collaboration with JBEI as part of the 2021 Laboratory Directed Research and Development (LDRD) program. Cole also received a DOE Early Career Research Program (ECRP) award in ...
Health Promotion and Chronic Disease Prevention in Canada: Research, Policy and Practice is seeking relevant topical research articles that present new findings or synthesize/review existing evidence on natural experiments of the built environment (or related policies) that influence health behaviours with implications for chronic disease ...
Abstract. This paper reviews a fungus - mushrooms. In this paper, identification, cultivation, uses, side effects, nutritional and medicinal values, storage, marketing and other uses of ...
The major research areas included: increasing maize yield, hybrid development, pests and diseases, irrigation, fertilization (nitrogen), drought, temperature, gene expression and climate change. The increasing number of published documents signifies an improved response to addressing maize production challenges through research in order to ...
Introduction. Onychomycosis is a fungal infection occurring in the nails and may affect the adjacent skin. Typically, it manifests as discoloration of the nail, nail plate thickening, and onycholysis 1.It is the most common nail pathology and accounts for about 90% of toenail infections worldwide 1.This infection presents several problems for affected populations, including local pain ...
Cellectis announced the publication of a new research paper in Molecular Therapy, demonstrating that TALEN-mediated intron editing of hematopoietic stem and progenitor cells enables transgene expression restricted to the myeloid lineage. ... This approach could unlock new therapeutic avenues for the treatment of inborn metabolic diseases as ...