- Open access
- Published: 19 July 2015
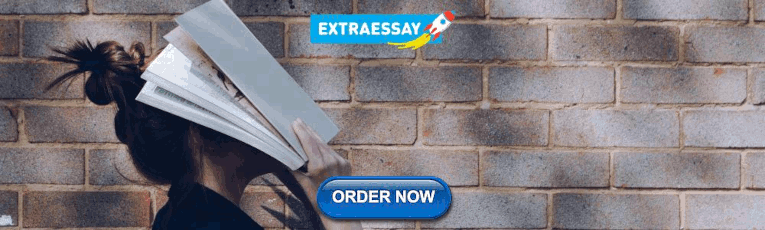
The role of visual representations in scientific practices: from conceptual understanding and knowledge generation to ‘seeing’ how science works
- Maria Evagorou 1 ,
- Sibel Erduran 2 &
- Terhi Mäntylä 3
International Journal of STEM Education volume 2 , Article number: 11 ( 2015 ) Cite this article
73k Accesses
78 Citations
13 Altmetric
Metrics details
The use of visual representations (i.e., photographs, diagrams, models) has been part of science, and their use makes it possible for scientists to interact with and represent complex phenomena, not observable in other ways. Despite a wealth of research in science education on visual representations, the emphasis of such research has mainly been on the conceptual understanding when using visual representations and less on visual representations as epistemic objects. In this paper, we argue that by positioning visual representations as epistemic objects of scientific practices, science education can bring a renewed focus on how visualization contributes to knowledge formation in science from the learners’ perspective.
This is a theoretical paper, and in order to argue about the role of visualization, we first present a case study, that of the discovery of the structure of DNA that highlights the epistemic components of visual information in science. The second case study focuses on Faraday’s use of the lines of magnetic force. Faraday is known of his exploratory, creative, and yet systemic way of experimenting, and the visual reasoning leading to theoretical development was an inherent part of the experimentation. Third, we trace a contemporary account from science focusing on the experimental practices and how reproducibility of experimental procedures can be reinforced through video data.
Conclusions
Our conclusions suggest that in teaching science, the emphasis in visualization should shift from cognitive understanding—using the products of science to understand the content—to engaging in the processes of visualization. Furthermore, we suggest that is it essential to design curriculum materials and learning environments that create a social and epistemic context and invite students to engage in the practice of visualization as evidence, reasoning, experimental procedure, or a means of communication and reflect on these practices. Implications for teacher education include the need for teacher professional development programs to problematize the use of visual representations as epistemic objects that are part of scientific practices.
During the last decades, research and reform documents in science education across the world have been calling for an emphasis not only on the content but also on the processes of science (Bybee 2014 ; Eurydice 2012 ; Duschl and Bybee 2014 ; Osborne 2014 ; Schwartz et al. 2012 ), in order to make science accessible to the students and enable them to understand the epistemic foundation of science. Scientific practices, part of the process of science, are the cognitive and discursive activities that are targeted in science education to develop epistemic understanding and appreciation of the nature of science (Duschl et al. 2008 ) and have been the emphasis of recent reform documents in science education across the world (Achieve 2013 ; Eurydice 2012 ). With the term scientific practices, we refer to the processes that take place during scientific discoveries and include among others: asking questions, developing and using models, engaging in arguments, and constructing and communicating explanations (National Research Council 2012 ). The emphasis on scientific practices aims to move the teaching of science from knowledge to the understanding of the processes and the epistemic aspects of science. Additionally, by placing an emphasis on engaging students in scientific practices, we aim to help students acquire scientific knowledge in meaningful contexts that resemble the reality of scientific discoveries.
Despite a wealth of research in science education on visual representations, the emphasis of such research has mainly been on the conceptual understanding when using visual representations and less on visual representations as epistemic objects. In this paper, we argue that by positioning visual representations as epistemic objects, science education can bring a renewed focus on how visualization contributes to knowledge formation in science from the learners’ perspective. Specifically, the use of visual representations (i.e., photographs, diagrams, tables, charts) has been part of science and over the years has evolved with the new technologies (i.e., from drawings to advanced digital images and three dimensional models). Visualization makes it possible for scientists to interact with complex phenomena (Richards 2003 ), and they might convey important evidence not observable in other ways. Visual representations as a tool to support cognitive understanding in science have been studied extensively (i.e., Gilbert 2010 ; Wu and Shah 2004 ). Studies in science education have explored the use of images in science textbooks (i.e., Dimopoulos et al. 2003 ; Bungum 2008 ), students’ representations or models when doing science (i.e., Gilbert et al. 2008 ; Dori et al. 2003 ; Lehrer and Schauble 2012 ; Schwarz et al. 2009 ), and students’ images of science and scientists (i.e., Chambers 1983 ). Therefore, studies in the field of science education have been using the term visualization as “the formation of an internal representation from an external representation” (Gilbert et al. 2008 , p. 4) or as a tool for conceptual understanding for students.
In this paper, we do not refer to visualization as mental image, model, or presentation only (Gilbert et al. 2008 ; Philips et al. 2010 ) but instead focus on visual representations or visualization as epistemic objects. Specifically, we refer to visualization as a process for knowledge production and growth in science. In this respect, modeling is an aspect of visualization, but what we are focusing on with visualization is not on the use of model as a tool for cognitive understanding (Gilbert 2010 ; Wu and Shah 2004 ) but the on the process of modeling as a scientific practice which includes the construction and use of models, the use of other representations, the communication in the groups with the use of the visual representation, and the appreciation of the difficulties that the science phase in this process. Therefore, the purpose of this paper is to present through the history of science how visualization can be considered not only as a cognitive tool in science education but also as an epistemic object that can potentially support students to understand aspects of the nature of science.
Scientific practices and science education
According to the New Generation Science Standards (Achieve 2013 ), scientific practices refer to: asking questions and defining problems; developing and using models; planning and carrying out investigations; analyzing and interpreting data; using mathematical and computational thinking; constructing explanations and designing solutions; engaging in argument from evidence; and obtaining, evaluating, and communicating information. A significant aspect of scientific practices is that science learning is more than just about learning facts, concepts, theories, and laws. A fuller appreciation of science necessitates the understanding of the science relative to its epistemological grounding and the process that are involved in the production of knowledge (Hogan and Maglienti 2001 ; Wickman 2004 ).
The New Generation Science Standards is, among other changes, shifting away from science inquiry and towards the inclusion of scientific practices (Duschl and Bybee 2014 ; Osborne 2014 ). By comparing the abilities to do scientific inquiry (National Research Council 2000 ) with the set of scientific practices, it is evident that the latter is about engaging in the processes of doing science and experiencing in that way science in a more authentic way. Engaging in scientific practices according to Osborne ( 2014 ) “presents a more authentic picture of the endeavor that is science” (p.183) and also helps the students to develop a deeper understanding of the epistemic aspects of science. Furthermore, as Bybee ( 2014 ) argues, by engaging students in scientific practices, we involve them in an understanding of the nature of science and an understanding on the nature of scientific knowledge.
Science as a practice and scientific practices as a term emerged by the philosopher of science, Kuhn (Osborne 2014 ), refers to the processes in which the scientists engage during knowledge production and communication. The work that is followed by historians, philosophers, and sociologists of science (Latour 2011 ; Longino 2002 ; Nersessian 2008 ) revealed the scientific practices in which the scientists engage in and include among others theory development and specific ways of talking, modeling, and communicating the outcomes of science.
Visualization as an epistemic object
Schematic, pictorial symbols in the design of scientific instruments and analysis of the perceptual and functional information that is being stored in those images have been areas of investigation in philosophy of scientific experimentation (Gooding et al. 1993 ). The nature of visual perception, the relationship between thought and vision, and the role of reproducibility as a norm for experimental research form a central aspect of this domain of research in philosophy of science. For instance, Rothbart ( 1997 ) has argued that visualizations are commonplace in the theoretical sciences even if every scientific theory may not be defined by visualized models.
Visual representations (i.e., photographs, diagrams, tables, charts, models) have been used in science over the years to enable scientists to interact with complex phenomena (Richards 2003 ) and might convey important evidence not observable in other ways (Barber et al. 2006 ). Some authors (e.g., Ruivenkamp and Rip 2010 ) have argued that visualization is as a core activity of some scientific communities of practice (e.g., nanotechnology) while others (e.g., Lynch and Edgerton 1988 ) have differentiated the role of particular visualization techniques (e.g., of digital image processing in astronomy). Visualization in science includes the complex process through which scientists develop or produce imagery, schemes, and graphical representation, and therefore, what is of importance in this process is not only the result but also the methodology employed by the scientists, namely, how this result was produced. Visual representations in science may refer to objects that are believed to have some kind of material or physical existence but equally might refer to purely mental, conceptual, and abstract constructs (Pauwels 2006 ). More specifically, visual representations can be found for: (a) phenomena that are not observable with the eye (i.e., microscopic or macroscopic); (b) phenomena that do not exist as visual representations but can be translated as such (i.e., sound); and (c) in experimental settings to provide visual data representations (i.e., graphs presenting velocity of moving objects). Additionally, since science is not only about replicating reality but also about making it more understandable to people (either to the public or other scientists), visual representations are not only about reproducing the nature but also about: (a) functioning in helping solving a problem, (b) filling gaps in our knowledge, and (c) facilitating knowledge building or transfer (Lynch 2006 ).
Using or developing visual representations in the scientific practice can range from a straightforward to a complicated situation. More specifically, scientists can observe a phenomenon (i.e., mitosis) and represent it visually using a picture or diagram, which is quite straightforward. But they can also use a variety of complicated techniques (i.e., crystallography in the case of DNA studies) that are either available or need to be developed or refined in order to acquire the visual information that can be used in the process of theory development (i.e., Latour and Woolgar 1979 ). Furthermore, some visual representations need decoding, and the scientists need to learn how to read these images (i.e., radiologists); therefore, using visual representations in the process of science requires learning a new language that is specific to the medium/methods that is used (i.e., understanding an X-ray picture is different from understanding an MRI scan) and then communicating that language to other scientists and the public.
There are much intent and purposes of visual representations in scientific practices, as for example to make a diagnosis, compare, describe, and preserve for future study, verify and explore new territory, generate new data (Pauwels 2006 ), or present new methodologies. According to Latour and Woolgar ( 1979 ) and Knorr Cetina ( 1999 ), visual representations can be used either as primary data (i.e., image from a microscope). or can be used to help in concept development (i.e., models of DNA used by Watson and Crick), to uncover relationships and to make the abstract more concrete (graphs of sound waves). Therefore, visual representations and visual practices, in all forms, are an important aspect of the scientific practices in developing, clarifying, and transmitting scientific knowledge (Pauwels 2006 ).
Methods and Results: Merging Visualization and scientific practices in science
In this paper, we present three case studies that embody the working practices of scientists in an effort to present visualization as a scientific practice and present our argument about how visualization is a complex process that could include among others modeling and use of representation but is not only limited to that. The first case study explores the role of visualization in the construction of knowledge about the structure of DNA, using visuals as evidence. The second case study focuses on Faraday’s use of the lines of magnetic force and the visual reasoning leading to the theoretical development that was an inherent part of the experimentation. The third case study focuses on the current practices of scientists in the context of a peer-reviewed journal called the Journal of Visualized Experiments where the methodology is communicated through videotaped procedures. The three case studies represent the research interests of the three authors of this paper and were chosen to present how visualization as a practice can be involved in all stages of doing science, from hypothesizing and evaluating evidence (case study 1) to experimenting and reasoning (case study 2) to communicating the findings and methodology with the research community (case study 3), and represent in this way the three functions of visualization as presented by Lynch ( 2006 ). Furthermore, the last case study showcases how the development of visualization technologies has contributed to the communication of findings and methodologies in science and present in that way an aspect of current scientific practices. In all three cases, our approach is guided by the observation that the visual information is an integral part of scientific practices at the least and furthermore that they are particularly central in the scientific practices of science.
Case study 1: use visual representations as evidence in the discovery of DNA
The focus of the first case study is the discovery of the structure of DNA. The DNA was first isolated in 1869 by Friedrich Miescher, and by the late 1940s, it was known that it contained phosphate, sugar, and four nitrogen-containing chemical bases. However, no one had figured the structure of the DNA until Watson and Crick presented their model of DNA in 1953. Other than the social aspects of the discovery of the DNA, another important aspect was the role of visual evidence that led to knowledge development in the area. More specifically, by studying the personal accounts of Watson ( 1968 ) and Crick ( 1988 ) about the discovery of the structure of the DNA, the following main ideas regarding the role of visual representations in the production of knowledge can be identified: (a) The use of visual representations was an important part of knowledge growth and was often dependent upon the discovery of new technologies (i.e., better microscopes or better techniques in crystallography that would provide better visual representations as evidence of the helical structure of the DNA); and (b) Models (three-dimensional) were used as a way to represent the visual images (X-ray images) and connect them to the evidence provided by other sources to see whether the theory can be supported. Therefore, the model of DNA was built based on the combination of visual evidence and experimental data.
An example showcasing the importance of visual representations in the process of knowledge production in this case is provided by Watson, in his book The Double Helix (1968):
…since the middle of the summer Rosy [Rosalind Franklin] had had evidence for a new three-dimensional form of DNA. It occurred when the DNA 2molecules were surrounded by a large amount of water. When I asked what the pattern was like, Maurice went into the adjacent room to pick up a print of the new form they called the “B” structure. The instant I saw the picture, my mouth fell open and my pulse began to race. The pattern was unbelievably simpler than those previously obtained (A form). Moreover, the black cross of reflections which dominated the picture could arise only from a helical structure. With the A form the argument for the helix was never straightforward, and considerable ambiguity existed as to exactly which type of helical symmetry was present. With the B form however, mere inspection of its X-ray picture gave several of the vital helical parameters. (p. 167-169)
As suggested by Watson’s personal account of the discovery of the DNA, the photo taken by Rosalind Franklin (Fig. 1 ) convinced him that the DNA molecule must consist of two chains arranged in a paired helix, which resembles a spiral staircase or ladder, and on March 7, 1953, Watson and Crick finished and presented their model of the structure of DNA (Watson and Berry 2004 ; Watson 1968 ) which was based on the visual information provided by the X-ray image and their knowledge of chemistry.
X-ray chrystallography of DNA
In analyzing the visualization practice in this case study, we observe the following instances that highlight how the visual information played a role:
Asking questions and defining problems: The real world in the model of science can at some points only be observed through visual representations or representations, i.e., if we are using DNA as an example, the structure of DNA was only observable through the crystallography images produced by Rosalind Franklin in the laboratory. There was no other way to observe the structure of DNA, therefore the real world.
Analyzing and interpreting data: The images that resulted from crystallography as well as their interpretations served as the data for the scientists studying the structure of DNA.
Experimenting: The data in the form of visual information were used to predict the possible structure of the DNA.
Modeling: Based on the prediction, an actual three-dimensional model was prepared by Watson and Crick. The first model did not fit with the real world (refuted by Rosalind Franklin and her research group from King’s College) and Watson and Crick had to go through the same process again to find better visual evidence (better crystallography images) and create an improved visual model.
Example excerpts from Watson’s biography provide further evidence for how visualization practices were applied in the context of the discovery of DNA (Table 1 ).
In summary, by examining the history of the discovery of DNA, we showcased how visual data is used as scientific evidence in science, identifying in that way an aspect of the nature of science that is still unexplored in the history of science and an aspect that has been ignored in the teaching of science. Visual representations are used in many ways: as images, as models, as evidence to support or rebut a model, and as interpretations of reality.
Case study 2: applying visual reasoning in knowledge production, the example of the lines of magnetic force
The focus of this case study is on Faraday’s use of the lines of magnetic force. Faraday is known of his exploratory, creative, and yet systemic way of experimenting, and the visual reasoning leading to theoretical development was an inherent part of this experimentation (Gooding 2006 ). Faraday’s articles or notebooks do not include mathematical formulations; instead, they include images and illustrations from experimental devices and setups to the recapping of his theoretical ideas (Nersessian 2008 ). According to Gooding ( 2006 ), “Faraday’s visual method was designed not to copy apparent features of the world, but to analyse and replicate them” (2006, p. 46).
The lines of force played a central role in Faraday’s research on electricity and magnetism and in the development of his “field theory” (Faraday 1852a ; Nersessian 1984 ). Before Faraday, the experiments with iron filings around magnets were known and the term “magnetic curves” was used for the iron filing patterns and also for the geometrical constructs derived from the mathematical theory of magnetism (Gooding et al. 1993 ). However, Faraday used the lines of force for explaining his experimental observations and in constructing the theory of forces in magnetism and electricity. Examples of Faraday’s different illustrations of lines of magnetic force are given in Fig. 2 . Faraday gave the following experiment-based definition for the lines of magnetic forces:
a Iron filing pattern in case of bar magnet drawn by Faraday (Faraday 1852b , Plate IX, p. 158, Fig. 1), b Faraday’s drawing of lines of magnetic force in case of cylinder magnet, where the experimental procedure, knife blade showing the direction of lines, is combined into drawing (Faraday, 1855, vol. 1, plate 1)
A line of magnetic force may be defined as that line which is described by a very small magnetic needle, when it is so moved in either direction correspondent to its length, that the needle is constantly a tangent to the line of motion; or it is that line along which, if a transverse wire be moved in either direction, there is no tendency to the formation of any current in the wire, whilst if moved in any other direction there is such a tendency; or it is that line which coincides with the direction of the magnecrystallic axis of a crystal of bismuth, which is carried in either direction along it. The direction of these lines about and amongst magnets and electric currents, is easily represented and understood, in a general manner, by the ordinary use of iron filings. (Faraday 1852a , p. 25 (3071))
The definition describes the connection between the experiments and the visual representation of the results. Initially, the lines of force were just geometric representations, but later, Faraday treated them as physical objects (Nersessian 1984 ; Pocovi and Finlay 2002 ):
I have sometimes used the term lines of force so vaguely, as to leave the reader doubtful whether I intended it as a merely representative idea of the forces, or as the description of the path along which the power was continuously exerted. … wherever the expression line of force is taken simply to represent the disposition of forces, it shall have the fullness of that meaning; but that wherever it may seem to represent the idea of the physical mode of transmission of the force, it expresses in that respect the opinion to which I incline at present. The opinion may be erroneous, and yet all that relates or refers to the disposition of the force will remain the same. (Faraday, 1852a , p. 55-56 (3075))
He also felt that the lines of force had greater explanatory power than the dominant theory of action-at-a-distance:
Now it appears to me that these lines may be employed with great advantage to represent nature, condition, direction and comparative amount of the magnetic forces; and that in many cases they have, to the physical reasoned at least, a superiority over that method which represents the forces as concentrated in centres of action… (Faraday, 1852a , p. 26 (3074))
For giving some insight to Faraday’s visual reasoning as an epistemic practice, the following examples of Faraday’s studies of the lines of magnetic force (Faraday 1852a , 1852b ) are presented:
(a) Asking questions and defining problems: The iron filing patterns formed the empirical basis for the visual model: 2D visualization of lines of magnetic force as presented in Fig. 2 . According to Faraday, these iron filing patterns were suitable for illustrating the direction and form of the magnetic lines of force (emphasis added):
It must be well understood that these forms give no indication by their appearance of the relative strength of the magnetic force at different places, inasmuch as the appearance of the lines depends greatly upon the quantity of filings and the amount of tapping; but the direction and forms of these lines are well given, and these indicate, in a considerable degree, the direction in which the forces increase and diminish . (Faraday 1852b , p.158 (3237))
Despite being static and two dimensional on paper, the lines of magnetic force were dynamical (Nersessian 1992 , 2008 ) and three dimensional for Faraday (see Fig. 2 b). For instance, Faraday described the lines of force “expanding”, “bending,” and “being cut” (Nersessian 1992 ). In Fig. 2 b, Faraday has summarized his experiment (bar magnet and knife blade) and its results (lines of force) in one picture.
(b) Analyzing and interpreting data: The model was so powerful for Faraday that he ended up thinking them as physical objects (e.g., Nersessian 1984 ), i.e., making interpretations of the way forces act. Of course, he made a lot of experiments for showing the physical existence of the lines of force, but he did not succeed in it (Nersessian 1984 ). The following quote illuminates Faraday’s use of the lines of force in different situations:
The study of these lines has, at different times, been greatly influential in leading me to various results, which I think prove their utility as well as fertility. Thus, the law of magneto-electric induction; the earth’s inductive action; the relation of magnetism and light; diamagnetic action and its law, and magnetocrystallic action, are the cases of this kind… (Faraday 1852a , p. 55 (3174))
(c) Experimenting: In Faraday's case, he used a lot of exploratory experiments; in case of lines of magnetic force, he used, e.g., iron filings, magnetic needles, or current carrying wires (see the quote above). The magnetic field is not directly observable and the representation of lines of force was a visual model, which includes the direction, form, and magnitude of field.
(d) Modeling: There is no denying that the lines of magnetic force are visual by nature. Faraday’s views of lines of force developed gradually during the years, and he applied and developed them in different contexts such as electromagnetic, electrostatic, and magnetic induction (Nersessian 1984 ). An example of Faraday’s explanation of the effect of the wire b’s position to experiment is given in Fig. 3 . In Fig. 3 , few magnetic lines of force are drawn, and in the quote below, Faraday is explaining the effect using these magnetic lines of force (emphasis added):
Picture of an experiment with different arrangements of wires ( a , b’ , b” ), magnet, and galvanometer. Note the lines of force drawn around the magnet. (Faraday 1852a , p. 34)
It will be evident by inspection of Fig. 3 , that, however the wires are carried away, the general result will, according to the assumed principles of action, be the same; for if a be the axial wire, and b’, b”, b”’ the equatorial wire, represented in three different positions, whatever magnetic lines of force pass across the latter wire in one position, will also pass it in the other, or in any other position which can be given to it. The distance of the wire at the place of intersection with the lines of force, has been shown, by the experiments (3093.), to be unimportant. (Faraday 1852a , p. 34 (3099))
In summary, by examining the history of Faraday’s use of lines of force, we showed how visual imagery and reasoning played an important part in Faraday’s construction and representation of his “field theory”. As Gooding has stated, “many of Faraday’s sketches are far more that depictions of observation, they are tools for reasoning with and about phenomena” (2006, p. 59).
Case study 3: visualizing scientific methods, the case of a journal
The focus of the third case study is the Journal of Visualized Experiments (JoVE) , a peer-reviewed publication indexed in PubMed. The journal devoted to the publication of biological, medical, chemical, and physical research in a video format. The journal describes its history as follows:
JoVE was established as a new tool in life science publication and communication, with participation of scientists from leading research institutions. JoVE takes advantage of video technology to capture and transmit the multiple facets and intricacies of life science research. Visualization greatly facilitates the understanding and efficient reproduction of both basic and complex experimental techniques, thereby addressing two of the biggest challenges faced by today's life science research community: i) low transparency and poor reproducibility of biological experiments and ii) time and labor-intensive nature of learning new experimental techniques. ( http://www.jove.com/ )
By examining the journal content, we generate a set of categories that can be considered as indicators of relevance and significance in terms of epistemic practices of science that have relevance for science education. For example, the quote above illustrates how scientists view some norms of scientific practice including the norms of “transparency” and “reproducibility” of experimental methods and results, and how the visual format of the journal facilitates the implementation of these norms. “Reproducibility” can be considered as an epistemic criterion that sits at the heart of what counts as an experimental procedure in science:
Investigating what should be reproducible and by whom leads to different types of experimental reproducibility, which can be observed to play different roles in experimental practice. A successful application of the strategy of reproducing an experiment is an achievement that may depend on certain isiosyncratic aspects of a local situation. Yet a purely local experiment that cannot be carried out by other experimenters and in other experimental contexts will, in the end be unproductive in science. (Sarkar and Pfeifer 2006 , p.270)
We now turn to an article on “Elevated Plus Maze for Mice” that is available for free on the journal website ( http://www.jove.com/video/1088/elevated-plus-maze-for-mice ). The purpose of this experiment was to investigate anxiety levels in mice through behavioral analysis. The journal article consists of a 9-min video accompanied by text. The video illustrates the handling of the mice in soundproof location with less light, worksheets with characteristics of mice, computer software, apparatus, resources, setting up the computer software, and the video recording of mouse behavior on the computer. The authors describe the apparatus that is used in the experiment and state how procedural differences exist between research groups that lead to difficulties in the interpretation of results:
The apparatus consists of open arms and closed arms, crossed in the middle perpendicularly to each other, and a center area. Mice are given access to all of the arms and are allowed to move freely between them. The number of entries into the open arms and the time spent in the open arms are used as indices of open space-induced anxiety in mice. Unfortunately, the procedural differences that exist between laboratories make it difficult to duplicate and compare results among laboratories.
The authors’ emphasis on the particularity of procedural context echoes in the observations of some philosophers of science:
It is not just the knowledge of experimental objects and phenomena but also their actual existence and occurrence that prove to be dependent on specific, productive interventions by the experimenters” (Sarkar and Pfeifer 2006 , pp. 270-271)
The inclusion of a video of the experimental procedure specifies what the apparatus looks like (Fig. 4 ) and how the behavior of the mice is captured through video recording that feeds into a computer (Fig. 5 ). Subsequently, a computer software which captures different variables such as the distance traveled, the number of entries, and the time spent on each arm of the apparatus. Here, there is visual information at different levels of representation ranging from reconfiguration of raw video data to representations that analyze the data around the variables in question (Fig. 6 ). The practice of levels of visual representations is not particular to the biological sciences. For instance, they are commonplace in nanotechnological practices:
Visual illustration of apparatus
Video processing of experimental set-up
Computer software for video input and variable recording
In the visualization processes, instruments are needed that can register the nanoscale and provide raw data, which needs to be transformed into images. Some Imaging Techniques have software incorporated already where this transformation automatically takes place, providing raw images. Raw data must be translated through the use of Graphic Software and software is also used for the further manipulation of images to highlight what is of interest to capture the (inferred) phenomena -- and to capture the reader. There are two levels of choice: Scientists have to choose which imaging technique and embedded software to use for the job at hand, and they will then have to follow the structure of the software. Within such software, there are explicit choices for the scientists, e.g. about colour coding, and ways of sharpening images. (Ruivenkamp and Rip 2010 , pp.14–15)
On the text that accompanies the video, the authors highlight the role of visualization in their experiment:
Visualization of the protocol will promote better understanding of the details of the entire experimental procedure, allowing for standardization of the protocols used in different laboratories and comparisons of the behavioral phenotypes of various strains of mutant mice assessed using this test.
The software that takes the video data and transforms it into various representations allows the researchers to collect data on mouse behavior more reliably. For instance, the distance traveled across the arms of the apparatus or the time spent on each arm would have been difficult to observe and record precisely. A further aspect to note is how the visualization of the experiment facilitates control of bias. The authors illustrate how the olfactory bias between experimental procedures carried on mice in sequence is avoided by cleaning the equipment.
Our discussion highlights the role of visualization in science, particularly with respect to presenting visualization as part of the scientific practices. We have used case studies from the history of science highlighting a scientist’s account of how visualization played a role in the discovery of DNA and the magnetic field and from a contemporary illustration of a science journal’s practices in incorporating visualization as a way to communicate new findings and methodologies. Our implicit aim in drawing from these case studies was the need to align science education with scientific practices, particularly in terms of how visual representations, stable or dynamic, can engage students in the processes of science and not only to be used as tools for cognitive development in science. Our approach was guided by the notion of “knowledge-as-practice” as advanced by Knorr Cetina ( 1999 ) who studied scientists and characterized their knowledge as practice, a characterization which shifts focus away from ideas inside scientists’ minds to practices that are cultural and deeply contextualized within fields of science. She suggests that people working together can be examined as epistemic cultures whose collective knowledge exists as practice.
It is important to stress, however, that visual representations are not used in isolation, but are supported by other types of evidence as well, or other theories (i.e., in order to understand the helical form of DNA, or the structure, chemistry knowledge was needed). More importantly, this finding can also have implications when teaching science as argument (e.g., Erduran and Jimenez-Aleixandre 2008 ), since the verbal evidence used in the science classroom to maintain an argument could be supported by visual evidence (either a model, representation, image, graph, etc.). For example, in a group of students discussing the outcomes of an introduced species in an ecosystem, pictures of the species and the ecosystem over time, and videos showing the changes in the ecosystem, and the special characteristics of the different species could serve as visual evidence to help the students support their arguments (Evagorou et al. 2012 ). Therefore, an important implication for the teaching of science is the use of visual representations as evidence in the science curriculum as part of knowledge production. Even though studies in the area of science education have focused on the use of models and modeling as a way to support students in the learning of science (Dori et al. 2003 ; Lehrer and Schauble 2012 ; Mendonça and Justi 2013 ; Papaevripidou et al. 2007 ) or on the use of images (i.e., Korfiatis et al. 2003 ), with the term using visuals as evidence, we refer to the collection of all forms of visuals and the processes involved.
Another aspect that was identified through the case studies is that of the visual reasoning (an integral part of Faraday’s investigations). Both the verbalization and visualization were part of the process of generating new knowledge (Gooding 2006 ). Even today, most of the textbooks use the lines of force (or just field lines) as a geometrical representation of field, and the number of field lines is connected to the quantity of flux. Often, the textbooks use the same kind of visual imagery than in what is used by scientists. However, when using images, only certain aspects or features of the phenomena or data are captured or highlighted, and often in tacit ways. Especially in textbooks, the process of producing the image is not presented and instead only the product—image—is left. This could easily lead to an idea of images (i.e., photos, graphs, visual model) being just representations of knowledge and, in the worse case, misinterpreted representations of knowledge as the results of Pocovi and Finlay ( 2002 ) in case of electric field lines show. In order to avoid this, the teachers should be able to explain how the images are produced (what features of phenomena or data the images captures, on what ground the features are chosen to that image, and what features are omitted); in this way, the role of visualization in knowledge production can be made “visible” to students by engaging them in the process of visualization.
The implication of these norms for science teaching and learning is numerous. The classroom contexts can model the generation, sharing and evaluation of evidence, and experimental procedures carried out by students, thereby promoting not only some contemporary cultural norms in scientific practice but also enabling the learning of criteria, standards, and heuristics that scientists use in making decisions on scientific methods. As we have demonstrated with the three case studies, visual representations are part of the process of knowledge growth and communication in science, as demonstrated with two examples from the history of science and an example from current scientific practices. Additionally, visual information, especially with the use of technology is a part of students’ everyday lives. Therefore, we suggest making use of students’ knowledge and technological skills (i.e., how to produce their own videos showing their experimental method or how to identify or provide appropriate visual evidence for a given topic), in order to teach them the aspects of the nature of science that are often neglected both in the history of science and the design of curriculum. Specifically, what we suggest in this paper is that students should actively engage in visualization processes in order to appreciate the diverse nature of doing science and engage in authentic scientific practices.
However, as a word of caution, we need to distinguish the products and processes involved in visualization practices in science:
If one considers scientific representations and the ways in which they can foster or thwart our understanding, it is clear that a mere object approach, which would devote all attention to the representation as a free-standing product of scientific labor, is inadequate. What is needed is a process approach: each visual representation should be linked with its context of production (Pauwels 2006 , p.21).
The aforementioned suggests that the emphasis in visualization should shift from cognitive understanding—using the products of science to understand the content—to engaging in the processes of visualization. Therefore, an implication for the teaching of science includes designing curriculum materials and learning environments that create a social and epistemic context and invite students to engage in the practice of visualization as evidence, reasoning, experimental procedure, or a means of communication (as presented in the three case studies) and reflect on these practices (Ryu et al. 2015 ).
Finally, a question that arises from including visualization in science education, as well as from including scientific practices in science education is whether teachers themselves are prepared to include them as part of their teaching (Bybee 2014 ). Teacher preparation programs and teacher education have been critiqued, studied, and rethought since the time they emerged (Cochran-Smith 2004 ). Despite the years of history in teacher training and teacher education, the debate about initial teacher training and its content still pertains in our community and in policy circles (Cochran-Smith 2004 ; Conway et al. 2009 ). In the last decades, the debate has shifted from a behavioral view of learning and teaching to a learning problem—focusing on that way not only on teachers’ knowledge, skills, and beliefs but also on making the connection of the aforementioned with how and if pupils learn (Cochran-Smith 2004 ). The Science Education in Europe report recommended that “Good quality teachers, with up-to-date knowledge and skills, are the foundation of any system of formal science education” (Osborne and Dillon 2008 , p.9).
However, questions such as what should be the emphasis on pre-service and in-service science teacher training, especially with the new emphasis on scientific practices, still remain unanswered. As Bybee ( 2014 ) argues, starting from the new emphasis on scientific practices in the NGSS, we should consider teacher preparation programs “that would provide undergraduates opportunities to learn the science content and practices in contexts that would be aligned with their future work as teachers” (p.218). Therefore, engaging pre- and in-service teachers in visualization as a scientific practice should be one of the purposes of teacher preparation programs.
Achieve. (2013). The next generation science standards (pp. 1–3). Retrieved from http://www.nextgenscience.org/ .
Google Scholar
Barber, J, Pearson, D, & Cervetti, G. (2006). Seeds of science/roots of reading . California: The Regents of the University of California.
Bungum, B. (2008). Images of physics: an explorative study of the changing character of visual images in Norwegian physics textbooks. NorDiNa, 4 (2), 132–141.
Bybee, RW. (2014). NGSS and the next generation of science teachers. Journal of Science Teacher Education, 25 (2), 211–221. doi: 10.1007/s10972-014-9381-4 .
Article Google Scholar
Chambers, D. (1983). Stereotypic images of the scientist: the draw-a-scientist test. Science Education, 67 (2), 255–265.
Cochran-Smith, M. (2004). The problem of teacher education. Journal of Teacher Education, 55 (4), 295–299. doi: 10.1177/0022487104268057 .
Conway, PF, Murphy, R, & Rath, A. (2009). Learning to teach and its implications for the continuum of teacher education: a nine-country cross-national study .
Crick, F. (1988). What a mad pursuit . USA: Basic Books.
Dimopoulos, K, Koulaidis, V, & Sklaveniti, S. (2003). Towards an analysis of visual images in school science textbooks and press articles about science and technology. Research in Science Education, 33 , 189–216.
Dori, YJ, Tal, RT, & Tsaushu, M. (2003). Teaching biotechnology through case studies—can we improve higher order thinking skills of nonscience majors? Science Education, 87 (6), 767–793. doi: 10.1002/sce.10081 .
Duschl, RA, & Bybee, RW. (2014). Planning and carrying out investigations: an entry to learning and to teacher professional development around NGSS science and engineering practices. International Journal of STEM Education, 1 (1), 12. doi: 10.1186/s40594-014-0012-6 .
Duschl, R., Schweingruber, H. A., & Shouse, A. (2008). Taking science to school . Washington DC: National Academies Press.
Erduran, S, & Jimenez-Aleixandre, MP (Eds.). (2008). Argumentation in science education: perspectives from classroom-based research . Dordrecht: Springer.
Eurydice. (2012). Developing key competencies at school in Europe: challenges and opportunities for policy – 2011/12 (pp. 1–72).
Evagorou, M, Jimenez-Aleixandre, MP, & Osborne, J. (2012). “Should we kill the grey squirrels?” A study exploring students’ justifications and decision-making. International Journal of Science Education, 34 (3), 401–428. doi: 10.1080/09500693.2011.619211 .
Faraday, M. (1852a). Experimental researches in electricity. – Twenty-eighth series. Philosophical Transactions of the Royal Society of London, 142 , 25–56.
Faraday, M. (1852b). Experimental researches in electricity. – Twenty-ninth series. Philosophical Transactions of the Royal Society of London, 142 , 137–159.
Gilbert, JK. (2010). The role of visual representations in the learning and teaching of science: an introduction (pp. 1–19).
Gilbert, J., Reiner, M. & Nakhleh, M. (2008). Visualization: theory and practice in science education . Dordrecht, The Netherlands: Springer.
Gooding, D. (2006). From phenomenology to field theory: Faraday’s visual reasoning. Perspectives on Science, 14 (1), 40–65.
Gooding, D, Pinch, T, & Schaffer, S (Eds.). (1993). The uses of experiment: studies in the natural sciences . Cambridge: Cambridge University Press.
Hogan, K, & Maglienti, M. (2001). Comparing the epistemological underpinnings of students’ and scientists’ reasoning about conclusions. Journal of Research in Science Teaching, 38 (6), 663–687.
Knorr Cetina, K. (1999). Epistemic cultures: how the sciences make knowledge . Cambridge: Harvard University Press.
Korfiatis, KJ, Stamou, AG, & Paraskevopoulos, S. (2003). Images of nature in Greek primary school textbooks. Science Education, 88 (1), 72–89. doi: 10.1002/sce.10133 .
Latour, B. (2011). Visualisation and cognition: drawing things together (pp. 1–32).
Latour, B, & Woolgar, S. (1979). Laboratory life: the construction of scientific facts . Princeton: Princeton University Press.
Lehrer, R, & Schauble, L. (2012). Seeding evolutionary thinking by engaging children in modeling its foundations. Science Education, 96 (4), 701–724. doi: 10.1002/sce.20475 .
Longino, H. E. (2002). The fate of knowledge . Princeton: Princeton University Press.
Lynch, M. (2006). The production of scientific images: vision and re-vision in the history, philosophy, and sociology of science. In L Pauwels (Ed.), Visual cultures of science: rethinking representational practices in knowledge building and science communication (pp. 26–40). Lebanon, NH: Darthmouth College Press.
Lynch, M. & S. Y. Edgerton Jr. (1988). ‘Aesthetic and digital image processing representational craft in contemporary astronomy’, in G. Fyfe & J. Law (eds), Picturing Power; Visual Depictions and Social Relations (London, Routledge): 184 – 220.
Mendonça, PCC, & Justi, R. (2013). An instrument for analyzing arguments produced in modeling-based chemistry lessons. Journal of Research in Science Teaching, 51 (2), 192–218. doi: 10.1002/tea.21133 .
National Research Council (2000). Inquiry and the national science education standards . Washington DC: National Academies Press.
National Research Council (2012). A framework for K-12 science education . Washington DC: National Academies Press.
Nersessian, NJ. (1984). Faraday to Einstein: constructing meaning in scientific theories . Dordrecht: Martinus Nijhoff Publishers.
Book Google Scholar
Nersessian, NJ. (1992). How do scientists think? Capturing the dynamics of conceptual change in science. In RN Giere (Ed.), Cognitive Models of Science (pp. 3–45). Minneapolis: University of Minnesota Press.
Nersessian, NJ. (2008). Creating scientific concepts . Cambridge: The MIT Press.
Osborne, J. (2014). Teaching scientific practices: meeting the challenge of change. Journal of Science Teacher Education, 25 (2), 177–196. doi: 10.1007/s10972-014-9384-1 .
Osborne, J. & Dillon, J. (2008). Science education in Europe: critical reflections . London: Nuffield Foundation.
Papaevripidou, M, Constantinou, CP, & Zacharia, ZC. (2007). Modeling complex marine ecosystems: an investigation of two teaching approaches with fifth graders. Journal of Computer Assisted Learning, 23 (2), 145–157. doi: 10.1111/j.1365-2729.2006.00217.x .
Pauwels, L. (2006). A theoretical framework for assessing visual representational practices in knowledge building and science communications. In L Pauwels (Ed.), Visual cultures of science: rethinking representational practices in knowledge building and science communication (pp. 1–25). Lebanon, NH: Darthmouth College Press.
Philips, L., Norris, S. & McNab, J. (2010). Visualization in mathematics, reading and science education . Dordrecht, The Netherlands: Springer.
Pocovi, MC, & Finlay, F. (2002). Lines of force: Faraday’s and students’ views. Science & Education, 11 , 459–474.
Richards, A. (2003). Argument and authority in the visual representations of science. Technical Communication Quarterly, 12 (2), 183–206. doi: 10.1207/s15427625tcq1202_3 .
Rothbart, D. (1997). Explaining the growth of scientific knowledge: metaphors, models and meaning . Lewiston, NY: Mellen Press.
Ruivenkamp, M, & Rip, A. (2010). Visualizing the invisible nanoscale study: visualization practices in nanotechnology community of practice. Science Studies, 23 (1), 3–36.
Ryu, S, Han, Y, & Paik, S-H. (2015). Understanding co-development of conceptual and epistemic understanding through modeling practices with mobile internet. Journal of Science Education and Technology, 24 (2-3), 330–355. doi: 10.1007/s10956-014-9545-1 .
Sarkar, S, & Pfeifer, J. (2006). The philosophy of science, chapter on experimentation (Vol. 1, A-M). New York: Taylor & Francis.
Schwartz, RS, Lederman, NG, & Abd-el-Khalick, F. (2012). A series of misrepresentations: a response to Allchin’s whole approach to assessing nature of science understandings. Science Education, 96 (4), 685–692. doi: 10.1002/sce.21013 .
Schwarz, CV, Reiser, BJ, Davis, EA, Kenyon, L, Achér, A, Fortus, D, et al. (2009). Developing a learning progression for scientific modeling: making scientific modeling accessible and meaningful for learners. Journal of Research in Science Teaching, 46 (6), 632–654. doi: 10.1002/tea.20311 .
Watson, J. (1968). The Double Helix: a personal account of the discovery of the structure of DNA . New York: Scribner.
Watson, J, & Berry, A. (2004). DNA: the secret of life . New York: Alfred A. Knopf.
Wickman, PO. (2004). The practical epistemologies of the classroom: a study of laboratory work. Science Education, 88 , 325–344.
Wu, HK, & Shah, P. (2004). Exploring visuospatial thinking in chemistry learning. Science Education, 88 (3), 465–492. doi: 10.1002/sce.10126 .
Download references
Acknowledgements
The authors would like to acknowledge all reviewers for their valuable comments that have helped us improve the manuscript.
Author information
Authors and affiliations.
University of Nicosia, 46, Makedonitissa Avenue, Egkomi, 1700, Nicosia, Cyprus
Maria Evagorou
University of Limerick, Limerick, Ireland
Sibel Erduran
University of Tampere, Tampere, Finland
Terhi Mäntylä
You can also search for this author in PubMed Google Scholar
Corresponding author
Correspondence to Maria Evagorou .
Additional information
Competing interests.
The authors declare that they have no competing interests.
Authors’ contributions
ME carried out the introductory literature review, the analysis of the first case study, and drafted the manuscript. SE carried out the analysis of the third case study and contributed towards the “Conclusions” section of the manuscript. TM carried out the second case study. All authors read and approved the final manuscript.
Rights and permissions
Open Access This article is distributed under the terms of the Creative Commons Attribution 4.0 International License ( https://creativecommons.org/licenses/by/4.0 ), which permits use, duplication, adaptation, distribution, and reproduction in any medium or format, as long as you give appropriate credit to the original author(s) and the source, provide a link to the Creative Commons license, and indicate if changes were made.
Reprints and permissions
About this article
Cite this article.
Evagorou, M., Erduran, S. & Mäntylä, T. The role of visual representations in scientific practices: from conceptual understanding and knowledge generation to ‘seeing’ how science works. IJ STEM Ed 2 , 11 (2015). https://doi.org/10.1186/s40594-015-0024-x
Download citation
Received : 29 September 2014
Accepted : 16 May 2015
Published : 19 July 2015
DOI : https://doi.org/10.1186/s40594-015-0024-x
Share this article
Anyone you share the following link with will be able to read this content:
Sorry, a shareable link is not currently available for this article.
Provided by the Springer Nature SharedIt content-sharing initiative
- Visual representations
- Epistemic practices
- Science learning
- Architecture and Design
- Asian and Pacific Studies
- Business and Economics
- Classical and Ancient Near Eastern Studies
- Computer Sciences
- Cultural Studies
- Engineering
- General Interest
- Geosciences
- Industrial Chemistry
- Islamic and Middle Eastern Studies
- Jewish Studies
- Library and Information Science, Book Studies
- Life Sciences
- Linguistics and Semiotics
- Literary Studies
- Materials Sciences
- Mathematics
- Social Sciences
- Sports and Recreation
- Theology and Religion
- Publish your article
- The role of authors
- Promoting your article
- Abstracting & indexing
- Publishing Ethics
- Why publish with De Gruyter
- How to publish with De Gruyter
- Our book series
- Our subject areas
- Your digital product at De Gruyter
- Contribute to our reference works
- Product information
- Tools & resources
- Product Information
- Promotional Materials
- Orders and Inquiries
- FAQ for Library Suppliers and Book Sellers
- Repository Policy
- Free access policy
- Open Access agreements
- Database portals
- For Authors
- Customer service
- People + Culture
- Journal Management
- How to join us
- Working at De Gruyter
- Mission & Vision
- De Gruyter Foundation
- De Gruyter Ebound
- Our Responsibility
- Partner publishers

Your purchase has been completed. Your documents are now available to view.
11. On the nature and role of visual representations in knowledge production and science communication
From the book science communication.
- Luc Pauwels
This chapter presents a conceptual framework for a more thorough and conscious investigation of visual representational practices within the different discourses of scientific data representation, conceptualization, and scholarly and public communication. While several scholars have pointed at the great diversity of visual representations and their uses, few systematic attempts have been made at devising a typology of uses or at producing an encompassing framework for increasing insight in this complex domain. Such a taxonomic attempt however may form the basis or starting point of a more conscious practice and an essential part of a program aimed at heightening both social and natural scientists’ visual literacy skills as well as those of science communicators.
- X / Twitter
Supplementary Materials
Please login or register with De Gruyter to order this product.
Chapters in this book (37)
Visual Representations of Knowledge for Strategy Communication
- Living reference work entry
- First Online: 09 February 2018
- Cite this living reference work entry
- Jianxin Ge 2 ,
- Sabrina Bresciani 3 &
- Hongjia Xu 2
201 Accesses
To convince employees to implement the organizational strategy is a pervasive issue in organizations: how can the communication of a corporate strategy be enhanced so that employees are committed to it? A recent experimental study found that visual representations can improve attitude toward the organizational strategy, and that a visual format improves employees’ intention to comply with the strategy only in the West but not in China. In order to explore the reasons why Westerner and Chinese subjects were found to have differing behaviors related to strategy commitment (despite having similar attitudes), we set up a qualitative study based on interviews with 51 managers in a Chinese company. The outcome suggests that Chinese employees have a slight preference for a visual format of strategy communication, but that they would comply with the strategy in any format it is presented - because it is the duty of the top managers to develop the strategy. These results differ substantially from Western employees, which need to be persuaded by the strategy to be committed to implement it.
This is a preview of subscription content, log in via an institution to check access.
Access this chapter
Institutional subscriptions
Adler NJ (1983) Cross-cultural management research: the ostrich and the trend. Acad Manag Rev 8(2):226–232
Google Scholar
Alexander LD (1985) Successfully implementing strategic decisions. Long Range Plan 18:91–97
Article Google Scholar
Ariely D (2008) Predictably irrational. HarperCollins, New York, p 20
Bresciani S, Eppler MJ (2009) The benefits of synchronous collaborative information visualization: evidence from an experimental evaluation. IEEE Trans Vis Comput Graph 15(6)
Bresciani S, Ge J, Yaru N (2014) Improving attitude toward corporate strategy with visual mapping: scale development and application in Europe and China. Front Bus Res China 8(4):480–499
Bruner J (1979) On knowing: essays for the left hand, 2nd edn. Harvard University Press, Boston
Comi A, Bischof N, Eppler MJ (2014) Beyond projection: using collaborative visualization to conduct qualitative interviews. Qual Res Organ Manag 9(2):110–133
Eppler MJ, Burkard RA (2007) Visual representations in knowledge management: framework and cases. J Knowl Manag 11:112–122
Eppler MJ, Ge J (2008) Communicating with diagrams: how intuitive and cross-cultural are business graphics? Euro Asia J Manag 18:3–22
Fenton EM (2007) Visualising strategic change: the role and impact of process maps as boundary objects in reorganisation. Eur Manag J 25(2):104–117
Kernbach S, Eppler MJ, Bresciani S (2014) The use of visualization in the communication of business strategies: an experimental evaluation. Int J Bus Commun. Sage 52(2):164–187
Li Y, Guo-hui S, Eppler MJ (2010) Making strategy work: a literature review on the factors influencing strategy implementation. In: Handbook of research on strategy process. Edward Elgar, Cheltenham, pp 165–183
Masuda T, Nisbett RE (2006) Culture and change blindness. Cogn Sci 30(2):381–399
Mintzberg H (1977) Strategy formulation as a historical process. Int Stud Manag Organ 7(2):28–40
Neher K (2013) Visual social media marketing: harnessing images, instagram, infographics and pinterest to grow your business online. Boot Camp Pub
Nisbett R (2003) The geography of thought: how Asians and Westerners think differently…and why. Free Press, New York
O’Donnell AM, Dansereau DF, Hall RH (2002) Knowledge maps as scaffolds for cognitive processing. Educ Psychol Rev 14(1):71–86
Peng W, Litteljohn D (2001) Organisational communication and strategy implementation – a primary inquiry. Int J Contemp Hosp Manag 13:360–363
Platts KW, Tan KH (2004) Strategy visualisation: knowing, understanding, and formulating. Manag Decis 42(5/6):667–676
Samovar L, Porter R, McDaniel E (2011) Intercultural communication: a reader. Cengage Learning, Boston
Shannon CE, Weaver W (1949) A mathematical model of communication. University of Urbana, Urbana
Snodgrass JG, Vanderwart M (1980) A standardized set of 260 pictures: norms for name agreement, image agreement, familiarity, and visual complexity. J Exp Psychol Hum Learn Mem 6(2):174–215
Sojka JZ, Giese JL (2006) Communicating through pictures and words: understanding the role of affect and cognition in processing visual and verbal information. Psychol Mark 23(12):995–1014
Suthers DD (2005) Collaborative knowledge construction through shared representations. In: Proceedings of the 38th Hawaii international conference on system sciences, (HICSS’05), Big Island, HI, USA
Tversky B (2005) Visuospatial reasoning. In: Holyoak K, Morrison R (eds) Handbook of reasoning. Cambridge University Press, Cambridge, pp 209–249
Wang P (2009) Strategic management: tools and cases. China Machine Press, Beijing
Yakura EK (2002) Charting time: timelines as temporal boundary objects. Acad Manag J 45(5):956–970
Download references
Author information
Authors and affiliations.
Business School, Central University of Finance and Economics, Beijing, China
Jianxin Ge & Hongjia Xu
Institute for Media and Communications Management – MCM, University of St. Gallen, St. Gallen, Switzerland
Sabrina Bresciani
You can also search for this author in PubMed Google Scholar
Corresponding author
Correspondence to Jianxin Ge .
Editor information
Editors and affiliations.
Sun Tzu Art of War Institute, University of St Andrews 1411 Sun Tzu Art of War Institute, Singapore, Singapore
Check-Teck Foo
Section Editor information
Appendix: three representations for a corporate strategy.
See Figs. 3 , 4 , and 5 .
Rights and permissions
Reprints and permissions
Copyright information
© 2018 Springer Nature Singapore Pte Ltd.
About this entry
Cite this entry.
Ge, J., Bresciani, S., Xu, H. (2018). Visual Representations of Knowledge for Strategy Communication. In: Foo, CT. (eds) Handbook of Chinese Management. Springer, Singapore. https://doi.org/10.1007/978-981-10-2442-9_2-1
Download citation
DOI : https://doi.org/10.1007/978-981-10-2442-9_2-1
Received : 09 December 2017
Accepted : 30 December 2017
Published : 09 February 2018
Publisher Name : Springer, Singapore
Print ISBN : 978-981-10-2442-9
Online ISBN : 978-981-10-2442-9
eBook Packages : Springer Reference Business and Management Reference Module Humanities and Social Sciences Reference Module Business, Economics and Social Sciences
- Publish with us
Policies and ethics
- Find a journal
- Track your research
To read this content please select one of the options below:
Please note you do not have access to teaching notes, visual representations in knowledge management: framework and cases.
Journal of Knowledge Management
ISSN : 1367-3270
Article publication date: 24 July 2007
The purpose of this article is to explore the potential of visualization for corporate knowledge management.
Design/methodology/approach
The employed methodology consists of a taxonomy of visualization formats that are embedded in a conceptual framework to guide the application of visualization in knowledge management according to the type of knowledge that is visualized, the knowledge management objective, the target group, and the application situation. This conceptual framework is illustrated through real‐life examples.
The findings show that there is much room for knowledge management applications based on visualization beyond the mere referencing of experts or documents through knowledge maps.
Research limitations/implications
The research implications thus consist of experimenting actively with new forms of visual knowledge representation and evaluating their benefits or potential drawbacks rigorously.
Practical implications
The authors encourage managers to look beyond simple diagrammatic representations of knowledge and explore alternative visual languages, such as visual metaphors or graphic narratives.
Originality/value
This paper consists of two elements: first, the systematic, descriptive and prescriptive approach towards visualization in knowledge management, and second the innovative examples of how to harness the power of visualization in knowledge management.
- Knowledge creation
- Knowledge transfer
Eppler, M.J. and Burkhard, R.A. (2007), "Visual representations in knowledge management: framework and cases", Journal of Knowledge Management , Vol. 11 No. 4, pp. 112-122. https://doi.org/10.1108/13673270710762756
Emerald Group Publishing Limited
Copyright © 2007, Emerald Group Publishing Limited
Related articles
We’re listening — tell us what you think, something didn’t work….
Report bugs here
All feedback is valuable
Please share your general feedback
Join us on our journey
Platform update page.
Visit emeraldpublishing.com/platformupdate to discover the latest news and updates
Questions & More Information
Answers to the most commonly asked questions here
REVIVE: Regional Visual Representation Matters in Knowledge-Based Visual Question Answering
Part of Advances in Neural Information Processing Systems 35 (NeurIPS 2022) Main Conference Track
Yuanze Lin, Yujia Xie, Dongdong Chen, Yichong Xu, Chenguang Zhu, Lu Yuan
This paper revisits visual representation in knowledge-based visual question answering (VQA) and demonstrates that using regional information in a better way can significantly improve the performance. While visual representation is extensively studied in traditional VQA, it is under-explored in knowledge-based VQA even though these two tasks share the common spirit, i.e., rely on visual input to answer the question. Specifically, we observe in most state-of-the-art knowledge-based VQA methods: 1) visual features are extracted either from the whole image or in a sliding window manner for retrieving knowledge, and the important relationship within/among object regions is neglected; 2) visual features are not well utilized in the final answering model, which is counter-intuitive to some extent. Based on these observations, we propose a new knowledge-based VQA method REVIVE, which tries to utilize the explicit information of object regions not only in the knowledge retrieval stage but also in the answering model. The key motivation is that object regions and inherent relationship are important for knowledge-based VQA. We perform extensive experiments on the standard OK-VQA dataset and achieve new state-of the-art performance, i.e., 58.0 accuracy, surpassing previous state-of-the-art method by a large margin (+3.6%). We also conduct detailed analysis and show the necessity of regional information in different framework components for knowledge-based VQA. Code is publicly available at https://github.com/yzleroy/REVIVE.
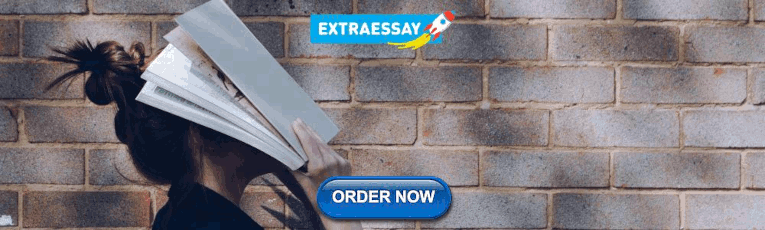
Name Change Policy
Requests for name changes in the electronic proceedings will be accepted with no questions asked. However name changes may cause bibliographic tracking issues. Authors are asked to consider this carefully and discuss it with their co-authors prior to requesting a name change in the electronic proceedings.
Use the "Report an Issue" link to request a name change.
VISTA : Visual-Textual Knowledge Graph Representation Learning
Jaejun Lee , Chanyoung Chung , Hochang Lee , Sungho Jo , Joyce Whang
Export citation
- Preformatted
Markdown (Informal)
[VISTA: Visual-Textual Knowledge Graph Representation Learning](https://aclanthology.org/2023.findings-emnlp.488) (Lee et al., Findings 2023)
- VISTA: Visual-Textual Knowledge Graph Representation Learning (Lee et al., Findings 2023)
- Jaejun Lee, Chanyoung Chung, Hochang Lee, Sungho Jo, and Joyce Whang. 2023. VISTA: Visual-Textual Knowledge Graph Representation Learning . In Findings of the Association for Computational Linguistics: EMNLP 2023 , pages 7314–7328, Singapore. Association for Computational Linguistics.
Suggestions or feedback?
MIT News | Massachusetts Institute of Technology
- Machine learning
- Social justice
- Black holes
- Classes and programs
Departments
- Aeronautics and Astronautics
- Brain and Cognitive Sciences
- Architecture
- Political Science
- Mechanical Engineering
Centers, Labs, & Programs
- Abdul Latif Jameel Poverty Action Lab (J-PAL)
- Picower Institute for Learning and Memory
- Lincoln Laboratory
- School of Architecture + Planning
- School of Engineering
- School of Humanities, Arts, and Social Sciences
- Sloan School of Management
- School of Science
- MIT Schwarzman College of Computing
Mapping the brain pathways of visual memorability
Press contact :.
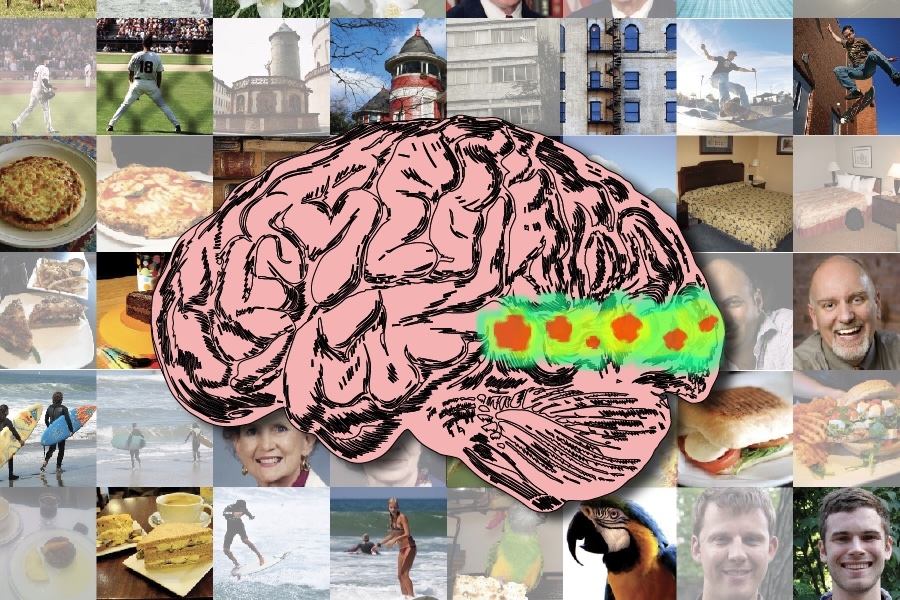
Previous image Next image
For nearly a decade, a team of MIT Computer Science and Artificial Intelligence Laboratory (CSAIL) researchers have been seeking to uncover why certain images persist in a people's minds, while many others fade. To do this, they set out to map the spatio-temporal brain dynamics involved in recognizing a visual image. And now for the first time, scientists harnessed the combined strengths of magnetoencephalography (MEG), which captures the timing of brain activity, and functional magnetic resonance imaging (fMRI), which identifies active brain regions, to precisely determine when and where the brain processes a memorable image.
Their open-access study, published this month in PLOS Biology , used 78 pairs of images matched for the same concept but differing in their memorability scores — one was highly memorable and the other was easy to forget. These images were shown to 15 subjects, with scenes of skateboarding, animals in various environments, everyday objects like cups and chairs, natural landscapes like forests and beaches, urban scenes of streets and buildings, and faces displaying different expressions. What they found was that a more distributed network of brain regions than previously thought are actively involved in the encoding and retention processes that underpin memorability.
“People tend to remember some images better than others, even when they are conceptually similar, like different scenes of a person skateboarding,” says Benjamin Lahner, an MIT PhD student in electrical engineering and computer science, CSAIL affiliate, and first author of the study. “We've identified a brain signature of visual memorability that emerges around 300 milliseconds after seeing an image, involving areas across the ventral occipital cortex and temporal cortex, which processes information like color perception and object recognition. This signature indicates that highly memorable images prompt stronger and more sustained brain responses, especially in regions like the early visual cortex, which we previously underestimated in memory processing.”
While highly memorable images maintain a higher and more sustained response for about half a second, the response to less memorable images quickly diminishes. This insight, Lahner elaborated, could redefine our understanding of how memories form and persist. The team envisions this research holding potential for future clinical applications, particularly in early diagnosis and treatment of memory-related disorders.
The MEG/fMRI fusion method, developed in the lab of CSAIL Senior Research Scientist Aude Oliva, adeptly captures the brain's spatial and temporal dynamics, overcoming the traditional constraints of either spatial or temporal specificity. The fusion method had a little help from its machine-learning friend, to better examine and compare the brain's activity when looking at various images. They created a “representational matrix,” which is like a detailed chart, showing how similar neural responses are in various brain regions. This chart helped them identify the patterns of where and when the brain processes what we see.
Picking the conceptually similar image pairs with high and low memorability scores was the crucial ingredient to unlocking these insights into memorability. Lahner explained the process of aggregating behavioral data to assign memorability scores to images, where they curated a diverse set of high- and low-memorability images with balanced representation across different visual categories.
Despite strides made, the team notes a few limitations. While this work can identify brain regions showing significant memorability effects, it cannot elucidate the regions' function in how it is contributing to better encoding/retrieval from memory.
“Understanding the neural underpinnings of memorability opens up exciting avenues for clinical advancements, particularly in diagnosing and treating memory-related disorders early on,” says Oliva. “The specific brain signatures we've identified for memorability could lead to early biomarkers for Alzheimer's disease and other dementias. This research paves the way for novel intervention strategies that are finely tuned to the individual's neural profile, potentially transforming the therapeutic landscape for memory impairments and significantly improving patient outcomes.”
“These findings are exciting because they give us insight into what is happening in the brain between seeing something and saving it into memory,” says Wilma Bainbridge, assistant professor of psychology at the University of Chicago, who was not involved in the study. “The researchers here are picking up on a cortical signal that reflects what's important to remember, and what can be forgotten early on.”
Lahner and Oliva, who is also the director of strategic industry engagement at the MIT Schwarzman College of Computing, MIT director of the MIT-IBM Watson AI Lab, and CSAIL principal investigator, join Western University Assistant Professor Yalda Mohsenzadeh and York University researcher Caitlin Mullin on the paper. The team acknowledges a shared instrument grant from the National Institutes of Health, and their work was funded by the Vannevar Bush Faculty Fellowship via an Office of Naval Research grant, a National Science Foundation award, Multidisciplinary University Research Initiative award via an Army Research Office grant, and the EECS MathWorks Fellowship. Their paper is published in PLOS Biology .
Share this news article on:
Related links.
- Project page
- Benjamin Lahner
- Computer Science and Artificial Intelligence Laboratory (CSAIL)
- Department of Electrical Engineering and Computer Science
Related Topics
- Brain and cognitive sciences
- Computer science and technology
- Artificial intelligence
- Image Processing
- Neuroscience
- Functional magnetic resonance imaging (fMRI)
- MIT-IBM Watson AI Lab
- Electrical Engineering & Computer Science (eecs)
Related Articles
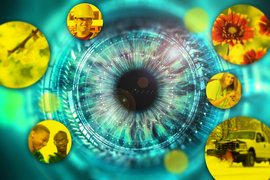
Researchers enhance peripheral vision in AI models
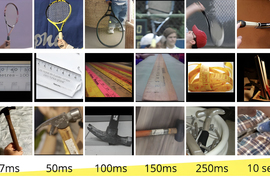
Image recognition accuracy: An unseen challenge confounding today’s AI
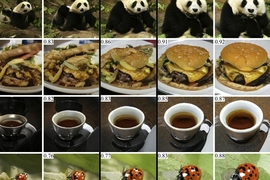
What makes an image memorable? Ask a computer
Previous item Next item
More MIT News
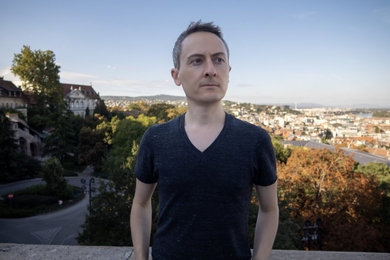
Creating bespoke programming languages for efficient visual AI systems
Read full story →
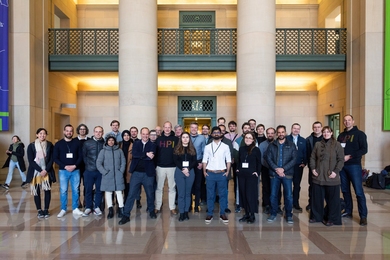
HPI-MIT design research collaboration creates powerful teams
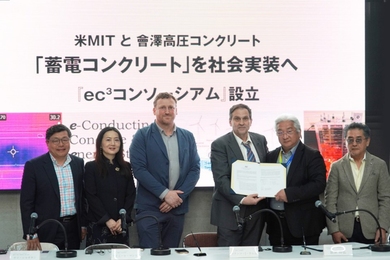
MIT conductive concrete consortium cements five-year research agreement with Japanese industry
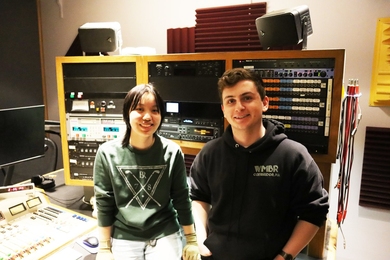
One of MIT’s best-kept secrets lives in the Institute’s basement
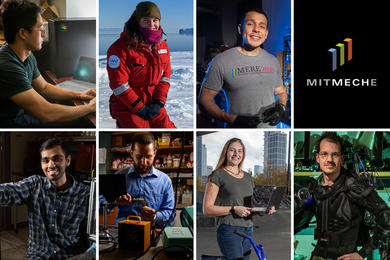
Exploring frontiers of mechanical engineering
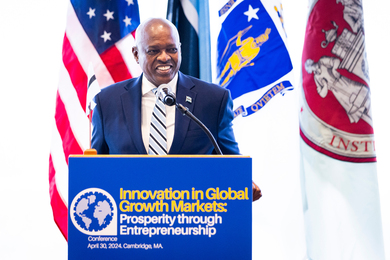
President Mokgweetsi Masisi of Botswana visits the Legatum Center at MIT
- More news on MIT News homepage →
Massachusetts Institute of Technology 77 Massachusetts Avenue, Cambridge, MA, USA
- Map (opens in new window)
- Events (opens in new window)
- People (opens in new window)
- Careers (opens in new window)
- Accessibility
- Social Media Hub
- MIT on Facebook
- MIT on YouTube
- MIT on Instagram
Help | Advanced Search
Quantitative Biology > Neurons and Cognition
Title: cocog: controllable visual stimuli generation based on human concept representations.
Abstract: A central question for cognitive science is to understand how humans process visual objects, i.e, to uncover human low-dimensional concept representation space from high-dimensional visual stimuli. Generating visual stimuli with controlling concepts is the key. However, there are currently no generative models in AI to solve this problem. Here, we present the Concept based Controllable Generation (CoCoG) framework. CoCoG consists of two components, a simple yet efficient AI agent for extracting interpretable concept and predicting human decision-making in visual similarity judgment tasks, and a conditional generation model for generating visual stimuli given the concepts. We quantify the performance of CoCoG from two aspects, the human behavior prediction accuracy and the controllable generation ability. The experiments with CoCoG indicate that 1) the reliable concept embeddings in CoCoG allows to predict human behavior with 64.07\% accuracy in the THINGS-similarity dataset; 2) CoCoG can generate diverse objects through the control of concepts; 3) CoCoG can manipulate human similarity judgment behavior by intervening key concepts. CoCoG offers visual objects with controlling concepts to advance our understanding of causality in human cognition. The code of CoCoG is available at \url{ this https URL }.
Submission history
Access paper:.
- HTML (experimental)
- Other Formats

References & Citations
- Google Scholar
- Semantic Scholar
BibTeX formatted citation

Bibliographic and Citation Tools
Code, data and media associated with this article, recommenders and search tools.
- Institution
arXivLabs: experimental projects with community collaborators
arXivLabs is a framework that allows collaborators to develop and share new arXiv features directly on our website.
Both individuals and organizations that work with arXivLabs have embraced and accepted our values of openness, community, excellence, and user data privacy. arXiv is committed to these values and only works with partners that adhere to them.
Have an idea for a project that will add value for arXiv's community? Learn more about arXivLabs .

© 2024 The Author(s)
This is an Open Access article distributed under the terms of the Creative Commons Attribution License ( http://creativecommons.org/licenses/by/4.0/ ), which permits unrestricted use, distribution, and reproduction in any medium, provided the original work is properly cited.
You are currently using an outdated browser. Click here to update to a newer version.

Idaho Falls Man Arrested for Obscene Visual Representation of the Sexual Abuse of Children
CATEGORY: ICAC
May 3, 2024
FOR IMMEDIATE RELEASE Media Contact: Dan Estes [email protected] 208-334-4112
Home Newsroom Idaho Falls Man Arrested for Obscene Visual Representation of the Sexual Abuse of Children
[ BOISE ] – Attorney General Raúl Labrador has announced investigators with his Idaho Internet Crimes Against Children (ICAC) Task Force arrested fifty-two-year-old Lloyd Perry on Wednesday, May 1st, 2024, for obscene visual representation of the sexual abuse of children.
The Bonneville County Sheriff’s Office, Idaho Falls Police Department, Rupert Police Department/K9, United States Marshal Service, and the United States Attorney’s Office for the District of Idaho assisted the Idaho ICAC Task Force with the arrest.
“This case involves thousands of AI-generated images of child sexual abuse,” said Attorney General Labrador. “Idaho’s recent law criminalizing AI images of child sexual abuse doesn’t go into effect until July 1 st , but this case spotlights the critical problem the law now addresses. I’m grateful for the hard work of our ICAC Unit and to the U.S. Attorney for the District of Idaho, whose team will be prosecuting this case federally.”
“Our office’s partnership with the ICAC Task Force of the Attorney General’s Office has a proven track-record of protecting Idaho’s children,” said U.S. Attorney Josh Hurwit. “As this investigation shows, we are fully committed to continuing our shared mission of identifying and prosecuting offenders who create, possess, or share child pornography. AI and other emerging technologies will never deter us.”
Anyone with information regarding the exploitation of children is encouraged to contact local police, the Attorney General’s ICAC Unit at 208-947-8700, or the National Center for Missing and Exploited Children at 1-800-843-5678.
The Attorney General’s ICAC Unit works with the Idaho ICAC Task Force, a coalition of federal, state, and local law enforcement agencies, to investigate and prosecute individuals who use the internet to criminally exploit children.
Parents, educators, and law enforcement officials can find more information and helpful resources at the ICAC website, ICACIdaho.org .
Idaho Falls Man Arrested for Obscene Visual Representation of the Sexual Abuse of Children

BOISE, Idaho (KMVT/KSVT) — Attorney General Raúl Labrador has announced investigators with his Idaho Internet Crimes Against Children (ICAC) Task Force arrested fifty-two-year-old Lloyd Perry on Wednesday, May 1, 2024, for obscene visual representation of the sexual abuse of children.
The Bonneville County Sheriff’s Office, Idaho Falls Police Department, Rupert Police Department/K9, United States Marshal Service, and the United States Attorney’s Office for the District of Idaho assisted the Idaho ICAC Task Force with the arrest.
“This case involves thousands of AI-generated images of child sexual abuse,” said Attorney General Labrador. “Idaho’s recent law criminalizing AI images of child sexual abuse doesn’t go into effect until July 1, but this case spotlights the critical problem the law now addresses. I’m grateful for the hard work of our ICAC Unit and to the U.S. Attorney for the District of Idaho, whose team will be prosecuting this case federally.”
“Our office’s partnership with the ICAC Task Force of the Attorney General’s Office has a proven track-record of protecting Idaho’s children,” said U.S. Attorney Josh Hurwit. “As this investigation shows, we are fully committed to continuing our shared mission of identifying and prosecuting offenders who create, possess, or share child pornography. AI and other emerging technologies will never deter us.”
Anyone with information regarding the exploitation of children is encouraged to contact local police, the Attorney General’s ICAC Unit at 208-947-8700, or the National Center for Missing and Exploited Children at 1-800-843-5678. The Attorney General’s ICAC Unit works with the Idaho ICAC Task Force, a coalition of federal, state, and local law enforcement agencies, to investigate and prosecute individuals who use the internet to criminally exploit children.
Parents, educators, and law enforcement officials can find more information and helpful resources at the ICAC website, ICACIdaho.org .
Copyright 2024 KMVT. All rights reserved.

Twin Falls Man Sentenced to Federal Prison for Providing Illegal Firearms, Including “Glock Switches,” to Californian with Cartel Ties
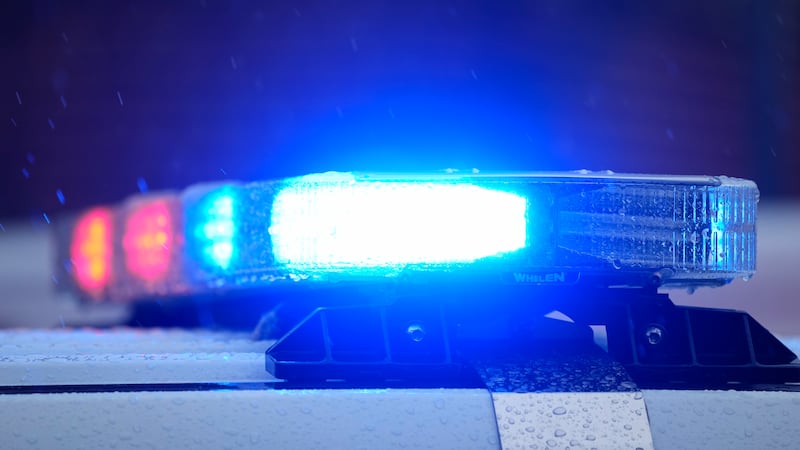
The Idaho State Police is investigating a fatal crash East of Burley
Birds to blame for power outage in Twin Falls
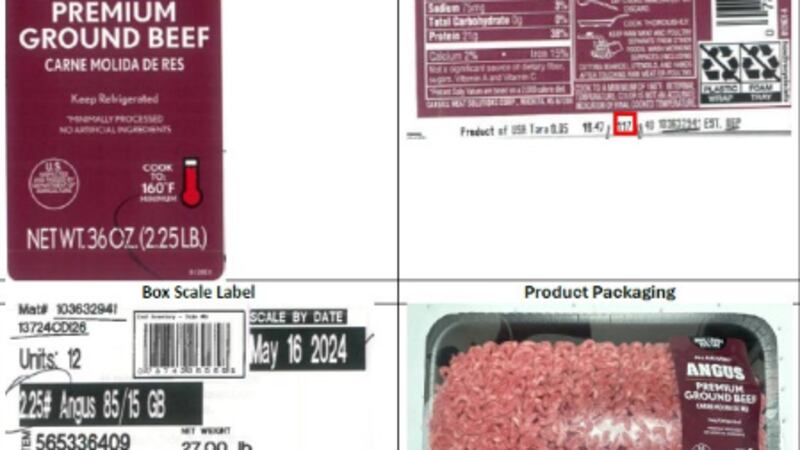
Walmart recalling 16k pounds of ground beef due to E. Coli contamination

Three Vandals and two Broncos earn NFL opportunities
Latest news.

Friday evening's online weather update {5/3/2024}

Pedestrian tips during national bike and youth traffic safety month

2 Twin Falls businesses team up to find homes for shelter animals
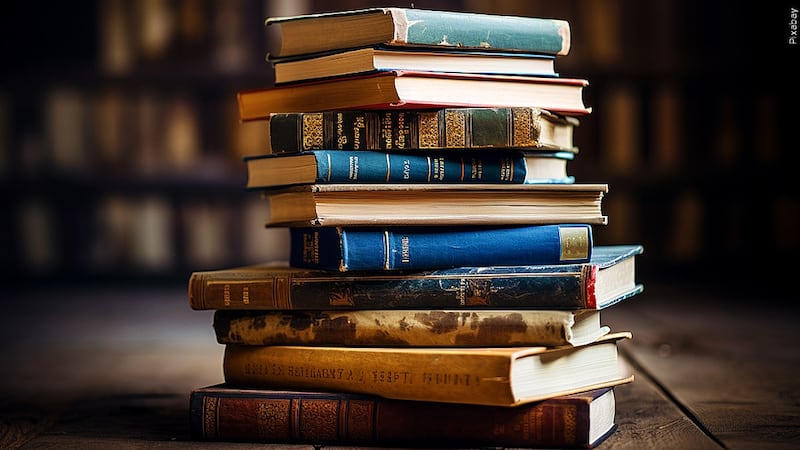
The evolution of reading a book

Illegal Ryan Davidson Yard Signs STILL Not Removed
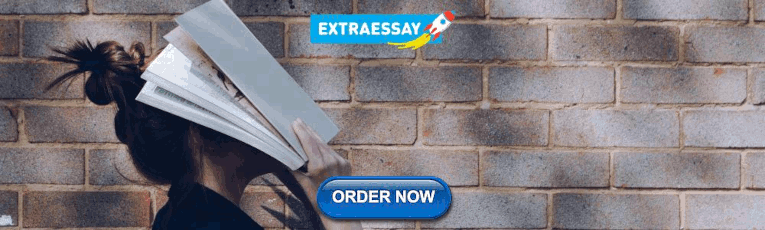
IMAGES
VIDEO
COMMENTS
The use of visual representations (i.e., photographs, diagrams, models) has been part of science, and their use makes it possible for scientists to interact with and represent complex phenomena, not observable in other ways. Despite a wealth of research in science education on visual representations, the emphasis of such research has mainly been on the conceptual understanding when using ...
Visual representations provide many particular advantages for learning, for example: ... When learners sketch actively, potentially beneficial cognitive processes include activation of prior knowledge, increased attention, and better subsequent recall as new information is integrated into long-term memory. When learners successfully retrieve ...
reasons why visual knowledge representations are used in knowledge management. Motives for knowledge visualization use are knowledge sharing through visual means, knowledge creation, learning from visual representations, codifying past experiences visually for future users, or mapping knowledge (Vail, 1999) so that experts, for example
Motives for knowledge visualization use are knowledge sharing through visual means, knowledge creation, learning from visual representations, codifying past experiences. visually for future users ...
Chemists routinely use visual representations to investigate relationships and move between the observable, physical level and the invisible particulate level (Kozma, Chin, Russell, ... Deconstructing and reconstructing knowledge and information in these ways has more generality than visual explanations: these techniques of analysis serve ...
On the nature and role of visual representations in knowledge production and science communication". Science Communication , edited by Annette Leßmöllmann, Marcelo Dascal and Thomas Gloning, Berlin, Boston: De Gruyter Mouton, 2020, pp. 235-256.
There is much room for knowledge management applications based on visualization beyond the mere referencing of experts or documents through knowledge maps, and the authors encourage managers to look beyond simple diagrammatic representations of knowledge and explore alternative visual languages, such as visual metaphors or graphic narratives. Purpose - The purpose of this article is to ...
Knowledge Visualization (KV), an essential part of knowledge mobilization, is a field of research that focuses on the creation and transfer of knowledge, specifically the use of visual representations to support and facilitate the communication of knowledge between at least two persons (Burkhard, Citation 2005; Meyer, Citation 2010).
Knowledge visualization within design thinking is the dynamic and interactive visualization of data as part of the non-direct and theoretical design thinking process. It can transfer crucial design data and strategies efficiently to practioners and researchers [4]. Nonetheless, not a lot of focus has been given to knowledge visualization as ...
The first one considered the importance of visual representations in science and its recent debate in education. It was already shown by philosophers of the Wiener Kreis that visual representation could serve for a better understanding and dissemination of knowledge to the broader public. As knowledge can be condensed in different non-verbal ...
Such influences of conceptual knowledge on perception may operate by altering the perceptual representation formed for novel objects and faces during training, or by recruiting top-down feedback from higher-order semantic to visual cortical areas, thus offsetting the perceptual demands of visual recognition (Bar et al., 2006). The latter ...
In their definition, knowledge visualization is a systematic approach which examines the use of visual representation to improve the management of knowledge in organizations. It "designates all graphic means that can be used to construct, assess measure, convey, or apply knowledge (i.e. complex insight, experiences, methods, etc.)" (Eppler ...
Visual representations may accentuate biases in decision making by increasing attention to particular attributes or less diagnostic information. High requirement on training and resources (Chen, 2005; van Wijk, 2006) The use of certain images or visual applications requires extensive training and support. Knowledge of visual conventions
Visual knowledge is a new form of knowledge representation that can encapsulate visual concepts and their relations in a succinct, com-prehensive, and interpretable manner, with a deep root in cognitive psychology. As the knowledge about the visual world has been iden-
Visu al information plays a fundamental role in our understanding, more than any other form of information (Colin, 2012). Colin (2012: 2) defines. visualisation as "a graphica l representation ...
processes. Prior knowledge can determine the ease with which learners can perceive and interpret visual representations in working memory. Although a long tradition of research has compared experts and novices, more research is necessary to fully explore the expert- novice continuum and maximize the potential of visual representations. 2006 ...
The idea of different representations having different potentials for communication and for providing access to disciplinary knowledge has been identified by some as "affordances" (Gibson, Citation 1979), or "disciplinary affordances" of a visual representation (Fredlund et al., Citation 2012), suggesting that different representations ...
Visual representations in knowledge management: framework and cases - Author: Martin J. Eppler, Remo A. Burkhard - The purpose of this article is to explore the potential of visualization for corporate knowledge management., - The employed methodology consists of a taxonomy of visualization formats that are embedded in a conceptual ...
This representation uses nested boxes rather than linked nodes to represent relations. The intricate structure of the knowledge base is conveyed by a combination of position, size, color, and font cues, MUE (Museum Unit Editor) was implemented using this representation to provide a graphic front end for the Cyc knowledge base.
This paper revisits visual representation in knowledge-based visual question answering (VQA) and demonstrates that using regional information in a better way can significantly improve the performance. While visual representation is extensively studied in traditional VQA, it is under-explored in knowledge-based VQA even though these two tasks ...
The use of visual representations (i.e., photographs, diagrams, models) has been part of science, and their use makes it possible for scientists to interact with and represent complex phenomena ...
We propose VISTA, a knowledge graph representation learning method for VTKGs, which incorporates the visual and textual representations of entities and relations using entity encoding, relation encoding, and triplet decoding transformers. Experiments show that VISTA outperforms state-of-the-art knowledge graph completion methods in real-world ...
A new method combining MEG and fMRI reveals how memorable images stimulate robust and enduring responses in the ventral visual cortex, enhancing our understanding of visual memorability and brain dynamics. ... where they curated a diverse set of high- and low-memorability images with balanced representation across different visual categories.
Artificial intelligence (AI)-based generative imaging systems such as DALL·E, Midjourney, Stable Diffusion, and Adobe Firefly, which work by transforming natural language descriptions into images, are revolutionizing computer vision. In this exploratory and qualitative research, we have replicated requests for images of women in different professions by comparing these representations in ...
A central question for cognitive science is to understand how humans process visual objects, i.e, to uncover human low-dimensional concept representation space from high-dimensional visual stimuli. Generating visual stimuli with controlling concepts is the key. However, there are currently no generative models in AI to solve this problem. Here, we present the Concept based Controllable ...
Despite literature critically examining trafficking representations, few studies have investigated visual representations of trafficking, or done so with reference to the national policy context wherein they are being utilized. This article explores visuals associated with trafficking in Italy with a focus on digital imagery retrieved via Google. The findings show that more "international ...
[BOISE] - Attorney General Raúl Labrador has announced investigators with his Idaho Internet Crimes Against Children (ICAC) Task Force arrested fifty-two-year-old Lloyd Perry on Wednesday, May 1st, 2024, for obscene visual representation of the sexual abuse of children.
Idaho Falls Man Arrested for Obscene Visual Representation of the Sexual Abuse of Children. BOISE, Idaho (KMVT/KSVT) — Attorney General Raúl Labrador has announced investigators with his Idaho ...
The image foundation model enables our scene tokens to encode the general knowledge of the open world while the LiDAR neural network encodes geometry information. Our proposed representation can efficiently encode the multi-frame multi-modality observations with a few hundred tokens and is compatible with most transformer-based architectures.