Dozens of COVID Virus Mutations Arose in Man With Longest Known Case
By Dennis Thompson HealthDay Reporter

FRIDAY, April 19, 2024 (HealthDay News) -- An immune-compromised man with a year-and-a-half-long COVID infection served as a breeding ground for dozens of coronavirus mutations, a new study discovered.
Worse, several of the mutations were in the COVID spike protein, indicating that the virus had attempted to evolve around current vaccines, researchers report.
“This case underscores the risk of persistent SARS-CoV-2 infections in immunocompromised individuals, as unique SARS-CoV-2 viral variants may emerge,” said the research team led by Magda Vergouwe . She's a doctoral candidate with Amsterdam University Medical Center in The Netherlands.
The patient in questioned endured the longest known COVID infection to date, fighting with the virus for 613 days before dying from the blood disease that had compromised his immune system, researchers said.
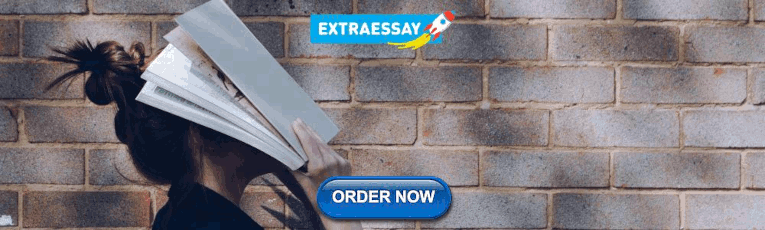
U.S. Cities With the Most Homelessness

Immune-compromised patients who suffer persistent infections give the COVID virus an opportunity to adapt and evolve, the investigators explained.
For instance, the Omicron variant is thought to have emerged in an immune-compromised patient initially infected with an earlier form of COVID, researchers said.
In this latest report, the man was admitted to Amsterdam University Medical Center in February 2022 with a COVID infection at age 72, after he’d already received multiple vaccinations.
He suffered from myelodysplastic and myeloproliferative overlap syndrome , a disease in which the bone marrow makes too many white blood cells, according to the U.S. National Cancer Institute.
Following a stem cell transplant, the man also had developed lymphoma, a cancer of the white blood cells, researchers said.
A drug he took for lymphoma, rituximab , depleted all the immune cells that normally produce antibodies for COVID, they added.
To clear his COVID, the man received a monoclonal antibody cocktail that ultimately proved ineffective.
In fact, gene sequencing showed that the coronavirus started mutating to evade the antibodies he’d received, a step that could have potentially undermined the effectiveness of the treatment in others, researchers said.
Gene sequencing of 27 nasal specimens taken from the man revealed more than 50 mutations in the COVID virus, including variants with changes in the spike protein targeted by vaccines.
“The prolonged infection has led to the emergence of a novel immune-evasive variant due to the extensive within-host evolution,” researchers said.
Such cases pose a “potential public health threat of possibly introducing viral escape variants into the community,” they added.
However, they noted that there had been no documented transmission of any COVID variants from the man into other people.
The researchers will present their findings at the European Society of Clinical Microbiology and Infectious Diseases meeting next week in Barcelona. Findings presented at medical meetings should be considered preliminary until published in a peer-reviewed journal.
More information
The U.S. Centers for Disease Control and Prevention has more about COVID .
SOURCE: European Society of Clinical Microbiology and Infectious Diseases, news release, April 19, 2024
Copyright © 2024 HealthDay . All rights reserved.
Join the Conversation
America 2024

Health News Bulletin
Stay informed on the latest news on health and COVID-19 from the editors at U.S. News & World Report.
Sign in to manage your newsletters »
Sign up to receive the latest updates from U.S News & World Report and our trusted partners and sponsors. By clicking submit, you are agreeing to our Terms and Conditions & Privacy Policy .
You May Also Like
The 10 worst presidents.
U.S. News Staff Feb. 23, 2024

Cartoons on President Donald Trump
Feb. 1, 2017, at 1:24 p.m.

Photos: Obama Behind the Scenes
April 8, 2022

Photos: Who Supports Joe Biden?
March 11, 2020
RFK Jr.: By the Numbers
Laura Mannweiler April 26, 2024

Biden’s Student Loan Chief to Step Down
Lauren Camera April 26, 2024

What to Know: Bird Flu Virus in Milk
Cecelia Smith-Schoenwalder April 26, 2024

Inflation a Stubborn Foe for the Fed
Tim Smart April 26, 2024

The Curse of the Modern Vice President

‘A Rule for the Ages’
Lauren Camera April 25, 2024

Real-Time Monitoring of Infectious Disease Outbreaks with a Combination of Google Trends Search Results and the Moving Epidemic Method: A Respiratory Syncytial Virus Case Study
Affiliations.
- 1 Health Economic and Decision Sciences, Merck & Co., Inc., Kenilworth, NJ 07065, USA.
- 2 Clinical Development, MSD, Kings Cross, London EC2M 6UR, UK.
- 3 Clinical Development, MSD, CH-6005 Luzern, Switzerland.
- 4 Clinical Development, Merck & Co., Inc., Kenilworth, NJ 07065, USA.
- 5 Health Economic and Decision Sciences, MSD, Kings Cross, London EC2M 6UR, UK.
- PMID: 36828491
- PMCID: PMC9962753
- DOI: 10.3390/tropicalmed8020075
The COVID-19 pandemic has disrupted the seasonal patterns of several infectious diseases. Understanding when and where an outbreak may occur is vital for public health planning and response. We usually rely on well-functioning surveillance systems to monitor epidemic outbreaks. However, not all countries have a well-functioning surveillance system in place, or at least not for the pathogen in question. We utilized Google Trends search results for RSV-related keywords to identify outbreaks. We evaluated the strength of the Pearson correlation coefficient between clinical surveillance data and online search data and applied the Moving Epidemic Method (MEM) to identify country-specific epidemic thresholds. Additionally, we established pseudo-RSV surveillance systems, enabling internal stakeholders to obtain insights on the speed and risk of any emerging RSV outbreaks in countries with imprecise disease surveillance systems but with Google Trends data. Strong correlations between RSV clinical surveillance data and Google Trends search results from several countries were observed. In monitoring an upcoming RSV outbreak with MEM, data collected from both systems yielded similar estimates of country-specific epidemic thresholds, starting time, and duration. We demonstrate in this study the potential of monitoring disease outbreaks in real time and complement classical disease surveillance systems by leveraging online search data.
Keywords: Google Trends; Moving Epidemic Method; RSV; epidemiology; nowcast; real-time.
Grants and funding
11 real and famous cases of malware attacks
- Updated at June 4, 2021
- Blog , Threat Research
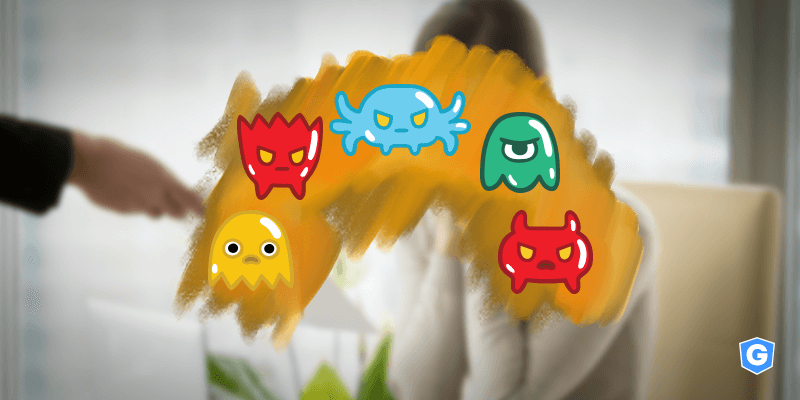
Many cases of famous hacker attacks use malware at some point. For example, first, the cybercriminal can send you a phishing email . No attachment. No links. Text only. After he gains your trust , in a second moment, he can send you a malicious attachment , that is, malware disguised as a legitimate file.
Malware is a malicious software designed to infect computers and other devices. The intent behind the infection varies. Why? Because the cybercriminal can use malware to make money, to steal secret information that can give strategic advantages, to prevent a business from running or even just to have fun.
Yes, there are hackers who act for pleasure.
In fact, malware is a broad term. It’s like a category. Within this category are different types of threats, such as virus , worm , trojan , and ransomware .
To fight malware delivered via email, here at Gatefy we offer a secure email gateway solution and an anti-fraud solution based on DMARC . You can request a demo or more information .
To get an idea, according to the FBI , damages caused by ransomware amounted to more than USD 29.1 million just in 2020. And one of the most widely used form of malware spreading continues to be via email . As a Verizon report confirmed : 30% of the malware was directly installed by the actor, 23% was sent there by email and 20% was dropped from a web application.
The cases listed below show how malware attacks can work and give you a glimpse of the harm they cause to businesses and individuals.
In this post, we’ll cover the following malware cases:
Table of Contents
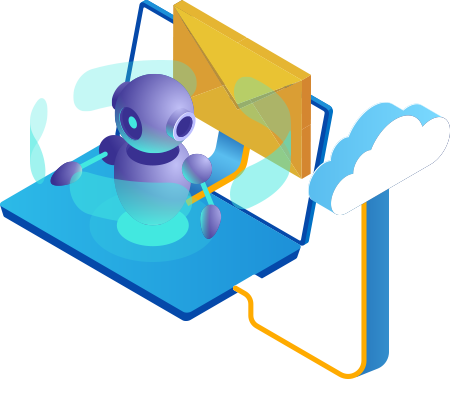
Check out 11 real cases of malware attacks
1. covidlock, ransomware, 2020.
Fear in relation to the Coronavirus (COVID-19) has been widely exploited by cybercriminals. CovidLock ransomware is an example. This type of ransomware infects victims via malicious files promising to offer more information about the disease.
The problem is that, once installed, CovidLock encrypts data from Android devices and denies data access to victims. To be granted access, you must pay a ransom of USD 100 per device.
2. LockerGoga, ransomware, 2019
LockerGoga is a ransomware that hit the news in 2019 for infecting large corporations in the world, such as Altran Technologies and Hydro. It’s estimated that it caused millions of dollars in damage in advanced and targeted attacks.
LockerGoga infections involve malicious emails , phishing scams and also credentials theft. LockerGoga is considered a very dangerous threat because it completely blocks victims’ access to the system.
3. Emotet, trojan, 2018
Emotet is a trojan that became famous in 2018 after the U.S. Department of Homeland Security defined it as one of the most dangerous and destructive malware. The reason for so much attention is that Emotet is widely used in cases of financial information theft, such as bank logins and cryptocurrencies.
The main vectors for Emotet’s spread are malicious emails in the form of spam and phishing campaigns . 2 striking examples are the case of the Chilean bank Consorcio, with damages of USD 2 million, and the case of the city of Allentown, Pennsylvania, with losses of USD 1 million.
4. WannaCry, ransomware, 2017
One of the worst ransomware attacks in history goes by the name of WannaCry , introduced via phishing emails in 2017. The threat exploits a vulnerability in Windows.
It’s estimated that more than 200,000 people have been reached worldwide by WannaCry, including hospitals, universities and large companies, such as FedEx, Telefonica, Nissan and Renault. The losses caused by WannaCry exceed USD 4 billion.
By the way, have you seen our article about the 7 real and famous cases of ransomware attacks ?
5. Petya, ransomware, 2016
Unlike most ransomware , Petya acts by blocking the machine’s entire operating system. We mean, Windows system. To release it, the victim has to pay a ransom.
It’s estimated that the losses involving Petya and its more new and destructive variations amount to USD 10 billion since it was released in 2016. Among the victims are banks, airports and oil and shipping companies from different parts of the world.
6. CryptoLocker, ransomware, 2013
The CryptoLocker is one of the most famous ransomware in history because, when it was released in 2013, it used a very large encryption key, which made the experts’ work difficult. It’s believed that it has caused more than USD 3 million in damage, infecting more than 200,000 Windows systems.
This type of ransomware was mainly distributed via emails, through malicious files that looked like PDF files , but, obviously, weren’t.
7. Stuxnet, worm, 2010
The Stuxnet deserves special mention on this list for being used in a political attack, in 2010, on Iran’s nuclear program and for exploiting numerous Windows zero-day vulnerabilities . This super-sophisticated worm has the ability to infect devices via USB drives, so there is no need for an internet connection.
Once installed, the malware is responsible for taking control of the system. It’s believed that it has been developed at the behest of some government. Read: USA and Israel.
8. Zeus, trojan, 2007
Zeus is a trojan distributed through malicious files hidden in emails and fake websites, in cases involving phishing . It’s well known for propagating quickly and for copying keystrokes, which led it to be widely used in cases of credential and passwords theft, such as email accounts and bank accounts.
The Zeus attacks hit major companies such as Amazon, Bank of America and Cisco. The damage caused by Zeus and its variations is estimated at more than USD 100 million since it was created in 2007.
9. MyDoom, worm, 2004
In 2004, the MyDoom worm became known and famous for trying to hit major technology companies, such as Google and Microsoft. It used to be spread by email using attention-grabbing subjects, such as “Error”, “Test” and “Mail Delivery System”.
MyDoom was used for DDoS attacks and as a backdoor to allow remote control. The losses are estimated, according to reports, in millions of dollars.
10. ILOVEYOU, worm, 2000
The ILOVEYOU worm was used to disguise itself as a love letter, received via email. Reports say that it infected more than 45 million people in the 2000s, causing more than USD 15 billion in damages.
ILOVEYOU is also considered as one of the first cases of social engineering used in malware attacks. Once executed, it had the ability to self-replicate using the victim’s email.
Also see 10 real and famous cases of social engineering .
11. Melissa, virus, 1999
The Melissa virus infected thousands of computers worldwide by the end of 1999. The threat was spread by email, using a malicious Word attachment and a catchy subject: “Important Message from (someone’s name)”.
Melissa is considered one of the earliest cases of social engineering in history. The virus had the ability to spread automatically via email. Reports from that time say that it infected many companies and people, causing losses estimated at USD 80 million.
How to fight malware attacks
There are 2 important points or fronts to fight and prevent infections caused by malware.
1. Cybersecurity awareness
The first point is the issue regarding cybersecurity awareness. You need to be aware on the internet. That means: watch out for suspicious websites and emails . And that old tip continues: if you’re not sure what you’re doing, don’t click on the links and don’t open attachments.
2. Technology to fight malware
The second point involves the use of technology . It’s important that you have an anti-malware solution on your computer or device. For end-users, there are several free and good options on the market.
For companies, in addition to this type of solution, we always recommend strengthening the protection of your email network. As already explained, email is the main malware vector. So, an email security solution can rid your business of major headaches.
Here at Gatefy we offer an email gateway solution and a DMARC solution . By the way, you can request a demo by clicking here or ask for more information . Our team of cybersecurity experts will contact you shortly to help.
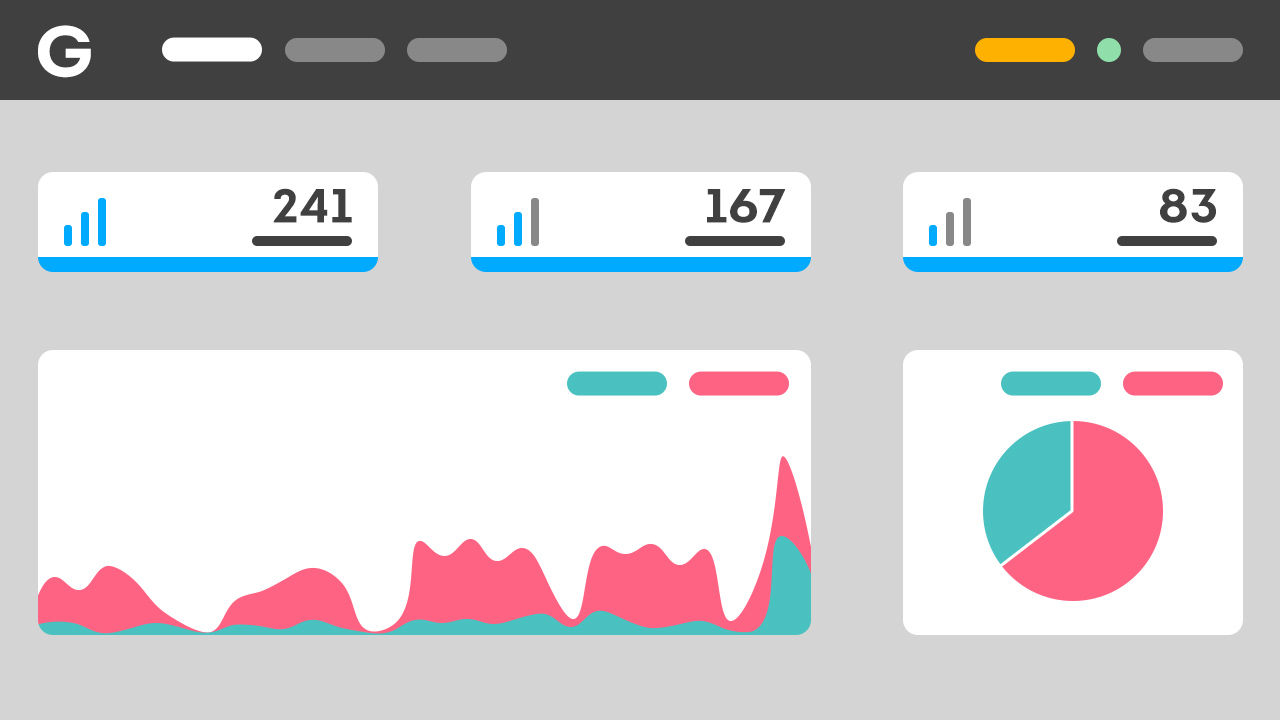
Latest news
10 real and famous cases of bec (business email compromise), 8 reasons to use dmarc in your business, what is mail server.
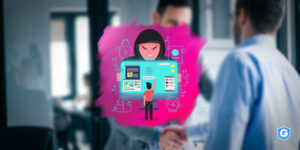
Influenza: A case study
Introduction
Most people have suffered from influenza (flu) at some time in their lives, so you probably have personal experience or a good idea of the symptoms and progression of the disease. However, influenza is actually one of the world’s most serious diseases. The pandemic of flu that occurred in 1918, immediately following the First World War, is thought to have killed up to 50 million people: many more than died in the war itself. More recently, the 2009 ‘swine flu’ pandemic caused widespread panic across parts of the world, although it resulted in relatively few fatalities.
The following course is a case study of influenza that considers a range of topics such as the nature of the virus itself, its spread, treatment, and diagnosis. There are activities to complete and videos to watch as you work through the text, and a set of self-assessment questions at the end of the course that allow you to judge how well you have understood the course content.
This OpenLearn course is an adapted extract from the Open University course : SK320 Infectious disease and public health .
Learning outcomes
After studying this course, you should be able to:
define and use, or recognise definitions and applications of, each of the glossary terms in the course
describe influenza viruses, their structure, how they are transmitted, how they infect cells and replicate and how they produce their damage in the host
outline the different types of immune defence which are deployed against flu infections, distinguishing those that act against infected cells from those that act against free virus
describe how strains of the virus change over time, and relate this to the flu viruses that occur in birds and other mammals
explain how the epidemic pattern of influenza can be related to the evolution of new strains of virus and to the specificity of the immune response against each strain.
1 Background to the case study
Influenza is a myxovirus belonging to the family of viruses known as Orthomyxoviridae. The virus was originally confined to aquatic birds, but it made the transition to humans 6000–9000 years ago, coinciding with the rise of farming, animal husbandry and urbanisation.
These changes in human behavior and population density provided the ecological niche that enabled influenza, as well as a number of other infectious agents such as the viruses that cause measles and smallpox, to move from animals and adapt to a human host.
Influenza as a disease has been recognised for centuries, even though the viruses which cause it were not correctly identified until the early 1930s, first in the UK and then in the USA. Indeed the name itself is derived from an Italian word meaning ‘influence’, and reflected the widespread belief in medieval times that the disease was caused by an evil climatic influence due to an unfortunate alignment of the stars.
Our current understanding – that infectious diseases are caused by infectious agents – is so ingrained that such mystical causes for an illness now seem absurd. However, even during the Middle Ages, people had a sound idea of infection and realised that some diseases could be passed from one individual to another and others could not. For example, the use of quarantine for a disease such as plague, but not for many other illnesses, shows that people could distinguish infectious diseases from non-infectious diseases even if the causative agent and the method of transmission were obscure.
The idea that influenza is caused by the influence of the stars, though not a satisfactory explanation of how the disease spread, does identify an important feature of flu – that serious epidemics of the disease occur at irregular intervals.
For example, in the twentieth century there were at least five major epidemics of flu that spread around the world (a pandemic), and there were less serious epidemics in most years. In times when people believed in the spontaneous generation of life, the stars would have seemed a reasonable explanation for unpleasant and unexpected epidemics.
1.1 Defining influenza
How would you define ‘influenza’?
You may well have defined influenza as an infection caused by an influenza virus. However, you may have defined it according to its symptoms: an infection that starts in the upper respiratory tract, with coughing and sneezing, spreads to give aching joints and muscles, and produces a fever that makes you feel awful; but usually it has gone in 5–10 days and most people make a full recovery.
The first answer here is the biological definition and, in the Open University course SK320, diseases are defined according to the infectious agent which produces them. This is because different infections can produce the same symptoms, and the same infectious agent can produce quite different symptoms in different people, depending on their age, genetic make-up or the tissue of the body that becomes infected. Here a distinction is made between the infectious disease caused by a particular agent and the disease symptoms.
Unfortunately there is a lot of confusion in common parlance about different diseases. Often, people say that they have ‘a bit of flu’ when they have an infection with some other virus, or a bacterium that produces flu-like symptoms. Such loose terminology is understandable, since most people are firstly concerned with the symptoms of their disease. But to treat and control disease requires accurate identification of the causative agent, so this is the starting point for considering any infectious disease.
Attributing cause to a disease
The difficulties encountered in assigning a particular pathogen to a disease are well-illustrated by influenza.
During the influenza pandemic that occurred in 1890, the microbiologist Pfeiffer isolated a novel bacterium from the lungs of people who had died of flu. The bacterium was named Haemophilus influenzae and since it was the only bacterium that could be regularly cultivated from these individuals at autopsy, it was assumed that H. influenzae was the causative agent of flu.
Again, in the 1918 flu pandemic, the bacterium could be regularly cultivated from people who had died of flu with pneumonia. So it was thought that flu was caused by the bacterium, and H. influenzae came to be called the ‘influenza bacillus’.
The role of H. influenzae was only brought into question in the early 1930s, when Smith, Andrews and Laidlaw showed that it was possible to transfer a flu-like illness from the nasal washings of an infected person to ferrets, using a bacteria-free filtrate. These studies demonstrated that the pathogen was in fact much smaller than any known bacterium and paved the way to the identification of influenza viruses (Figure 1).

Electron micrograph picture of influenza viruses. Each viral particle is about 500 nm across and surrounded by a darker-staining lipid bilayer coat derived from the host cell.
Why do you suppose that H. influenzae was incorrectly identified as the causative agent of flu?
The bacterium fulfils two of Koch’s postulates: it is regularly found in serious flu infections and it can be cultured in pure form on artificial media. Moreover, at that time no-one knew what a virus was, and everyone was thinking in terms of bacterial causes for infectious diseases.
Although the precise role of H. influenzae in the 1890 and 1918 flu pandemics is not clear, it is likely that the bacteria were present and acting in concert with the flu virus to produce the pneumonia experienced. Such synergy between virus and bacteria was demonstrated by Shope in 1931. He infected pigs with a bacterial-free filtrate (containing swine influenza virus) with or without the bacteria, and showed that the disease produced by the bacteria and filtrate together was more severe than that produced by either one alone (Van Epps, 2006).
In its role of co-pathogen, H. influenzae is only one of a number of bacteria that can exacerbate the viral infection. This highlights a very important point. In the tidy world of a microbiology or immunology laboratory, scientists typically examine the effect of one infectious agent in producing disease. In the real world, people often become infected with more than one pathogen. Indeed, infection with one agent often lays a person open to infection with another, as immune defences become overwhelmed. For this reason, a particular disease as seen by physicians may be due to a combination of pathogens.
1.2 Influenza infection in humans
Influenza is an acute viral disease that affects the respiratory tract in humans. The virus is spread readily in aerosol droplets produced by coughing and sneezing, which are symptoms of the illness. Other symptoms include fatigue, muscle and joint pains and fever.
Following infection, the influenza virus replicates in the cells lining the host’s upper and lower respiratory tract. Virus production peaks 1–2 days later, and virus particles are shed in secretions over the following 3–4 days. During this period, the patient is infectious and the symptoms are typically at their most severe.
After one week, virus is no longer produced, although it is possible to detect viral antigens for up to 2 weeks. Immune responses are initiated immediately after the virus starts to replicate, and antibodies against the virus start to appear in the blood at 3–4 days post infection. These continue to increase over the following days and persist in the blood for many months.
In a typical flu infection, the virus is completely eliminated from the host’s system within 2 weeks. This is sterile immunity: the virus cannot be obtained from the patient after recovery from the disease. Figure 2 shows the typical time course of an acute flu infection.

In this figure, the horizontal axis is labelled days and is marked from zero, which is the time of infection, up to 10 days at one-day intervals. There are three horizontal bars, labelled virus, symptoms and antibodies, and the depth of each bar corresponds to the amount of virus or antibody or the severity of symptoms respectively. Antibody production is maximal by day 5 and the virus is reduced to a very low level by day 6. Symptoms of infection disappear by day 9.
For infants, older people, and those with other underlying diseases (e.g. of the heart or respiratory system) an infection with flu may prove fatal. However, the severity of a flu epidemic and the case fatality rate depend on the strain of flu involved and the level of immunity in the host population.
During a severe epidemic, there are typically thousands more deaths than would normally be expected for that time of year, and these can be attributed to the disease. Although older people are usually most at risk from fatal disease, this is not always so. In the 1918 flu pandemic there was a surprisingly high death rate in people aged 20–40 (Figure 3), and this was also the case for the 2009 ‘swine flu’ pandemic.
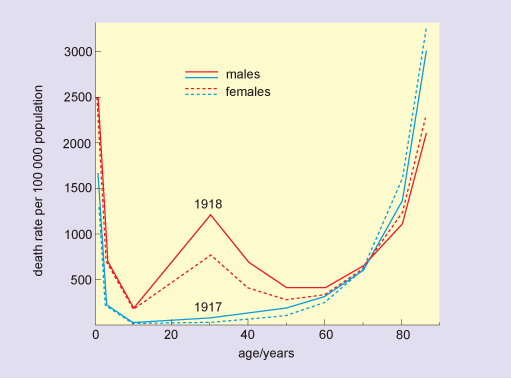
The figure is a line graph in which the horizontal axis is labelled age and is marked in years from zero to 80 at intervals of 20 years. The vertical axis is labelled death rate per 100 000 population and is marked from zero to 3000 at intervals of 500 units. The 1917 plots for both males and females are almost identical U-shaped curves, and show that death rates were in excess of 1500 in newborns, dropped to 200 by age 2 and were zero in 10-year-olds. Rates remained below 200 for those aged 40 or under, increased to 500 in 65-year-olds, and beyond this age, death rate increased very rapidly to reach levels of 3000 per 100 000 for 80-year-olds. The pattern in death rates in 1918 for the younger and older age groups was similar to that in 1917, although rates in newborns were much higher, at 2500. The minimum rate in 1918, again observed in 10-year-olds, was 200. Beyond this age, death rates rose sharply, to 1200 for 30-year-old males and 750 for 30-year-old females. Death rates in both groups fell rapidly, and for those aged 60 or more, were similar to the rates in the previous year.
Older people are often most severely affected during infectious disease outbreaks because they may have a less effective immune response than younger people, or a reduced capacity to repair and regenerate tissue damaged by the infection. However, there are circumstances where older people may be more resistant to infection than younger people because they may have already encountered the disease (in their youth) and could retain some immunity and so be less susceptible than younger people who have not encountered the disease before.
1.3 Influenza infection in other species
Influenza viruses infect a wide range of species, including pigs, horses, ducks, chickens and seals. In most of these other species the virus produces an acute infection.
For example, in most of the mammals the symptoms are very similar to those in humans: an acute infection of the respiratory tract, which is controlled by the immune response although fatal infections occur in some species. However, in wild ducks and other aquatic birds the virus primarily infects the gut and the birds do not appear to have any physical symptoms.
Despite this, ducks may remain infected for 2–4 weeks and during this time they shed virus in their faeces. Potentially this is a very important reservoir of infection; although flu viruses do not often cross the species barrier, the pool of viruses present in other species is an important genetic reservoir for the generation of new flu viruses that do infect humans.
This reservoir becomes particularly important in certain farming communities or in crowded conditions where animals (especially pigs and ducks) are continuously in close proximity with humans (Branswell, 2010). Although such conditions occur in many agricultural communities throughout the world, they are typically observed in South-East and East Asia thereby contributing to these geographical areas often being the source of radically new ‘hybrid’ strains of influenza that incorporate genes from different species-specific strains. (The genetics of influenza are discussed in Section 2.3.)
When strategies for controlling a disease are considered, awareness of the possible presence of an animal reservoir of infection is very important. For example, an immunisation programme against flu would substantially reduce the incidence of the current strain in humans but, because there is always a reservoir of these viruses in other animals, and these viruses are constantly mutating, another strain would inevitably emerge and be unaffected by immunisation. It is useful to distinguish diseases such as rabies, which primarily affect other vertebrates and occasionally infect humans (zoonoses), from diseases such as flu where different strains of the virus can affect several species including humans.
Identify a fundamental difference between the way that zoonoses (e.g. rabies) are transmitted, and the way in which flu is transmitted.
Flu can be transmitted from one human being to another, whereas most zoonoses, including rabies, are not transmitted between people.
2 Influenza viruses
Viruses have very diverse genomes. Whereas the genomes of bacteria, plants and animals are of double-stranded DNA, the genomes of viruses can be constituted from either DNA or RNA and may be double- or single-stranded molecules.
Usually, DNA is a double-stranded molecule with paired, complementary strands (dsDNA) and RNA is a single stranded molecule (ssRNA). However, some viruses have single-stranded DNA genomes (ssDNA) and some have double-stranded RNA genomes (dsRNA). The type of nucleic acid found in the genome depends on the group of viruses involved.
RNA encodes protein in all living things, and the sequence of bases in the RNA determines the sequence of amino acids in the protein. A strand of RNA which has the potential to encode protein is said to be ‘positive sense’ (+). If a strand of RNA is complementary to this, then it is ‘negative sense’ (–). Negative-sense RNA must first be copied to a complementary positive-sense strand of RNA before it can be translated into protein.
The description of the influenza genome as negative-sense ssRNA means that its RNA cannot be translated without copying first. This copying is performed by influenza’s viral RNA polymerase, a small amount of which is packaged with the virus, ready to begin copying the viral genome once it enters a host cell. Viral RNA polymerase consists of three subunits: PB1, PB2, and PA, encoded separately by the first three viral RNA strands.
Understanding the way in which different viruses replicate is important, since it allows the identification of particular points in their life-cycle that may be susceptible to treatment with antiviral drugs.
Classification
Viruses are classified into different families, groups and subgroups in much the same way as are species of animals or plants.
As you have already read, the influenza viruses are (–)ssRNA organisms (Baltimore group V) and belong to a family called the Orthomyxoviruses (see Box 1). They fall into three groups: influenza A, B and C.
Type A viruses are able to infect a wide variety of endothermic (warm-blooded) animals, including mammals and birds, and analysis of their viral genome indicates that all strains of influenza A originated from aquatic birds.
By contrast, types B and C are mostly confined to humans. At any one time, a number of different strains of virus may be circulating in the human population.
Box 1 Families, groups and strains of virus
Viruses were originally classified into different groups according to similarities in their structure, mode of replication and disease symptoms. For example, the Orthomyxoviruses include viruses that cause different types of influenza, while Paramyxoviruses include the viruses that cause measles and mumps.
Such large groupings are often called a family of viruses. The families can be subdivided into smaller groups, such as influenza A, B and C. Even within a single such group of viruses there can be an enormous level of genetic diversity, and this is the basis of the different strains. As an example, two HIV particles from the same individual may be 4% different in their genome; compare this with the 1% difference between the genomes of humans and chimpanzees, which are different species.
2.1 Structure of influenza
The structure of influenza A is shown schematically in Figure 4. The viral genomic RNA, which consists of eight separate strands (see Section 2.3), is enclosed by its associated nucleoproteins to make a ribonucleoprotein complex (RNP), and this is contained in the central core of the virus (the capsid).
The nucleoproteins are required for viral replication and packing of the genome into the new capsid, which is formed by M1-protein (or matrix protein). The M1-protein is the most abundant component of the virus, constituting about 40% of the viral mass; it is essential for the structural integrity of the virus and to control assembly of the virus.
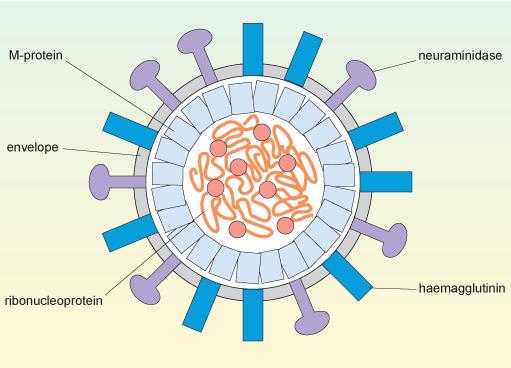
In this cross-sectional diagram of the spherical virus, the convoluted ribonucleoprotein genome with its replication enzymes is inside the capsid which comprises M-protein subunits. The envelope surrounds the capsid and is studded with molecules of haemagglutinin and neuraminidase.
Orthomyxoviruses have a capsid surrounded by a phospholipid bilayer derived from the plasma membrane of the cell that produced the virus. This layer is shown in Figure 4 as the virus’s envelope.
Two proteins, haemagglutinin and neuraminidase, are found on the viral envelope. These proteins are encoded by the viral HA and NA genes (Section 2.3), respectively and are inserted into the plasma membrane of the infected cell before the newly-produced viruses bud off from the cell surface.
The haemagglutinin can bind to glycophorin, a type of polysaccharide that contains sialic acid residues, and which is present on the surface of a variety of host cells. The virus uses the haemagglutinin to attach to the host cells that it will infect. Antibodies and drugs against haemagglutinin are therefore particularly important in limiting the spread of the virus, since they prevent it from attaching to new host cells.
Neuraminidase is an enzyme that cleaves sialic acid residues from polysaccharides. It has a role in clearing a path to the surface of the target cell before infection, namely, digesting the components of mucus surrounding epithelial cells in the respiratory system. Similarly, neuraminidase also promotes release of the budding virus from the cell surface after infection.
The structures of influenza B and influenza C are broadly similar to that of Type A, although in influenza C the functions of the haemagglutinin and the neuraminidase are combined in a single molecule, haemagglutinin esterase. This molecule binds and cleaves a less common type of sialic acid. Influenza C does not normally cause clinical disease or epidemics, so the following discussion is confined to influenza A and B.
2.2 Designation of strains of influenza
A considerable number of genetically different strains of influenza A have been identified, and these are classified according to where they were first isolated and according to the type of haemagglutinin and neuraminidase they express. For example ‘A/Shandong/9/93(H3N2)’ is an influenza A virus isolated in the Shandong province of China in 1993 – the ninth isolate in that year – and it has haemagglutinin type 3 and neuraminidase type 2.
At the start of the twenty-first century, the major circulating influenza A strains are H1N1 (‘swine flu’) and H3N2. At least 16 major variants of haemagglutinin and 9 variants of neuraminidase have been recognised, but to date most of these have only been found in birds.
The designation for influenza B is similar, but omits the information on the surface molecules, for example: ‘B/Panama/45/90’.
As you will see later, accurate identification of different strains of flu is crucial if we are to control epidemics by vaccination programmes.
2.3 Genomic diversity of influenza
The genome of flu viruses consists of around 14 000 nucleotides of negative-sense single-stranded RNA. Compare this number to the approximately 3 billion nucleotides found in the human genome or the 150 billion nucleotides of the genome of the marbled lungfish (the largest genome known in vertebrates).
The genome of influenza viruses is segmented, into eight distinct fragments of RNA containing 11 genes and encoding approximately 14 proteins (see Table 1 below). This structure has significance for the spread of the virus and the severity of disease symptoms.
Cases of influenza generally arise in two main ways: by provoking seasonal annual outbreaks or epidemics and, less commonly, through global pandemics. As you will see shortly, both of these phenomena occur as consequences of the fact that the virus uses RNA as its genetic template and that this RNA genome is segmented into discrete strands.
Footnotes
The influenza virus is a successful pathogen because it is constantly changing. How might having a segmented genome promote the evolution of new strains of influenza virus?
If a cell is infected with more than one strain of virus at the same time, then a new strain can be generated simply by mixing RNA strands from different viruses.
2.3.1 Creation of new viral strains
Part of the success of influenza as a pathogen is because segmented genome improves the virus’s potential to evolve into new strains through the combination of different RNA stands. This mixing of the genetic material from different viral strains to produce a new strain is termed genetic reassortment .
For instance, the virus that caused the 2009 H1N1 ‘swine flu’ pandemic comprises a quadruple reassortment of RNA strands from two swine virus, one avian virus, and one human influenza virus:
- the surface HA and NA proteins derive from two different swine influenzas (H1 from a North American swine influenza and N1 from a European swine influenza)
- the three components of the RNA polymerase derive from avian and human influenzas (PA and PB2 from the avian source, PB1 from the human 1993 H3N2 strain)
- the remaining internal proteins derive from the two swine influenzas (MacKenzie, 2009).
This does not necessarily mean that all four viruses infected the same animal at once. The new strain was likely the result of a reassortment of two swine influenza viruses, one from North America and one from Europe. The North American virus may itself have been the product of previous reassortments, containing a human PB1 gene since 1993 and an avian PA and PB2 genes since 2001. The presence of avian influenza RNA polymerase genes in this virus was especially worrying, since the avian polymerase is thought to be more efficient than human or swine versions, allowing the virus to replicate faster and thus making it more virulent. Similar avian RNA polymerase genes are what make H5N1 bird flu extremely virulent in mammals and what made the 1918 human pandemic virus so lethal in people.
This mixing of genes from two or more viruses (whether from the same host species or from different species) can cause major changes in the antigenic surface proteins of a virus, such that it is no longer recognised by the host’s immune system. This antigenic shift is described in more detail in Section 3 (specifically, Box 2).
In contrast to the major genetic changes caused by reassortment, influenza viruses also undergo constant, gradual, genetic changes due to errors made by their RNA polymerases.
2.4 Infection and replication
Influenza RNA polymerase lacks the ability to recognise and repair any errors that occur during genome duplication, resulting in mistakes in copying its viral RNA about once in every 10 000 nucleotides. Because the influenza genome only contains approximately 14 000 nucleotides, this means that, on average, each new virus produced differs by 1 or 2 nucleotides from its ‘parent’.
The slow accumulation of random genetic changes, especially in the antigenic surface proteins, explains why antibodies that were effective against the virus one year may be less effective against it in subsequent years. This gradual change in the nature of viral antigens is known as antigenic drift .
The replication cycle of influenza is illustrated in Figure 5.

Complicated diagram showing a series of events from the replication cycle of a flu virus, from the virus entering a cell, releasing its contents, replicating, and these new virus particles budding and exiting the infected cell. Anti-clockwise, from the top left corner: (1) virus attaches to cell and is endocytosed; (2) cellular lysosomes fuse with endocytosed virus and viral RNA, and proteins are released into cell; (3) viral RNA moves to nucleus of the infected cell and is transcribed into viral mRNA, which is exported into cytosol to make new viral proteins; (4) viral proteins and viral RNA self-combine to make new viral particles that bud off the cell and are released to infect more host cells.
Influenza is spread in aerosol droplets that contain virus particles (or by desiccated viral nuclei droplets), and infection may occur if these come into contact with the respiratory tract. Viral neuraminidase cleaves polysaccharides in the protective mucus coating the tract, which allows the virus to reach the surface of the respiratory epithelium.
The haemagglutinin now attaches to glycophorins (sialic-acid-containing glycoproteins) on the surface of the host cell, and the virus is taken up by endocytosis into a phagosome. Acidic lysosomes fuse with the phagosome to form a phagolysosome and the pH inside the phagolysosome falls. This promotes fusion of the viral envelope with the membrane of the phagolysosome, triggering uncoating of the viral capsid and release of viral RNA and nucleoproteins into the cytosol.
The viral genomic RNA then migrates to the nucleus where replication of the viral genome and transcription of viral mRNA occur. These processes require both host and viral enzymes. The viral negative-stranded RNA is replicated by the viral RNA-dependent RNA polymerase, into a positive-sense complementary RNA (cRNA), and these positive and negative RNA strands associate to form double-stranded RNA (dsRNA). The cRNA strand is subsequently replicated again to produce new viral genomic negative-stranded RNA. Some of the cRNA is also processed into mRNA for translation of viral proteins. The infection cycle is rapid and viral molecules can be detected inside the host cell within an hour of the initial infection.
The envelope glycoproteins (haemagglutinin and neuraminidase) are translated in the endoplasmic reticulum, processed and transported to the cell’s plasma membrane. The viral capsid is assembled within the nucleus of the infected cell. The capsid moves to the plasma membrane, where it buds off, taking a segment of membrane containing the haemagglutinin and neuraminidase, and this forms the new viral envelope.
Influenza virus budding from the surface of an infected cell is shown in Figure 6.
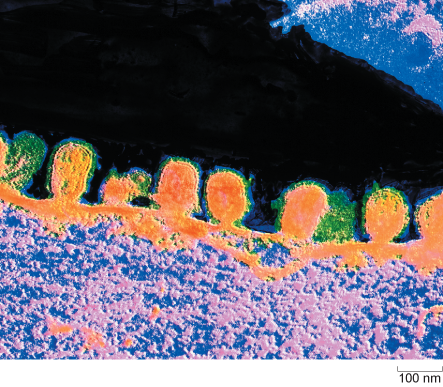
In this false-colour electron micrograph, the budding virus particles appear orange and their surrounding envelopes appear green. One of the buds has a short stalk which indicates that virus particle is about to pinch off from the host cell membrane. Each virus is about 100 nanometres in diameter.
From the description above, identify a process or element in the replication cycle which is characteristic of the virus, and which would not normally occur in a mammalian cell.
The replication of RNA on an RNA template with the production of double-stranded RNA would never normally occur in a mammalian cell. Double stranded RNA is therefore a signature of a viral infection. Significantly, cells have a way of detecting the presence of dsRNA, and this activates interferons: molecules involved in limiting viral replication.
2.5 Cellular pathology of influenza infection
Flu viruses can infect a number of different cell types from different species. This phenomenon is partly because the cellular glycoproteins which are recognised by viral haemagglutinin are widely distributed in the infectious agent.
What is the term for the property of viruses that allows them to only replicate in particular cell types?
This property is viral tropism. Hence we can say that flu viruses have a broad tropism.
A second reason why the virus can infect a variety of cell types is that the replication strategy of flu is relatively simple: ‘infect the cell, replicate as quickly as possible and then get out again’. This is the cytopathic effect of the virus. Cell death caused directly by the virus can be distinguished from cell death caused by the actions of the immune system as it eliminates infected cells.
The effects of cell death
Cell death impairs the function of an infected organ and often induces inflammation , a process that brings white cells (leukocytes) and molecules of the immune system to the site of infection. In the first instance, the leukocytes are involved in limiting the spread of infection; later they become involved in combating the infection, and in the final phase they clear cellular debris so that the tissue can repair or regenerate.
The symptoms of flu experienced by an infected person are partly due to the cytopathic effect of the virus, partly due to inflammation and partly a result of the innate immune response against the virus. The severity of the disease largely depends on the rate at which these processes occur.
- In most instances, the immune response develops sufficiently quickly to control the infection and patients recover.
- If viral replication and damage outstrip the development of the immune response then a fatal infection can occur.
In severe flu infections, the lungs may fill with fluid as the epithelium lining the alveoli (air sacs) is damaged by the virus. The fluid is ideal for the growth of bacteria, and this can lead to a bacterial pneumonia, in which the lungs become infected with one or more types of bacteria such as Haemophilus influenzae . Damage to cells lining blood vessels can cause local bleeding into the tissues, and this form of ‘fulminating disease’ was regularly seen in post-mortem lung tissues of people who died in the 1918 pandemic.
3 Patterns of disease
In humans, pigs and horses, flu viruses circulate through populations at regular intervals. The disease is endemic in tropical regions for all of these host groups (i.e. it is continually present in the community). In temperate latitudes, infections are usually seasonal or epidemic, with the greatest numbers occurring in the winter months (Figure 7). Epidemics also occur sporadically in sea mammals and poultry, and in these species high mortality is typical.

In this graph, the horizontal axis is marked in years from 1994 to 1997. The vertical axis is labelled number of isolates per week and is marked from zero to 900 at intervals of 100. The data show that there were three separate epidemics, which occurred over the three successive winters, and in increasing order of severity. The peak numbers of isolates were as follows: in 1994/5, there were 370; in 1995/6, there were 630; and in 1996/9, there were nearly 900.
In most years, flu in humans affects a minority of the population, the disease course is not very severe and the level of mortality is not great. In such years the influenza virus is slightly different from the previous year due to antigenic drift, which results in the accumulation of genetic mutations that cause the molecules present on the surface of the virus change progressively. In this scenario, the virus is not significantly different from the previous year so that the host’s immune system can more easily mount an effective response than it could to a completely new strain.
However, at irregular intervals the virus undergoes an antigenic shift. This process only occurs in influenza A viruses, typically every 10–30 years, and it is associated with severe pandemics, serious disease and high mortality (see Box 2).
In Section 2.3 you read that strains of influenza are differentiated and designated using a simple system of numbers and letters that depend on their surface antigens. More commonly, however, strains responsible for pandemics are often given a common name according to the area in the world from which they were thought to originate, or the species they mainly affected before becoming transferred to humans (see Table 2). Evidence suggests, however, that in the twentieth century the major flu pandemics all originated in China, with the exception of the 1918 pandemic, which first occurred in the USA.
Box 2 The rise of H5N1 – an example of antigenic shift
In 1997, a new strain of influenza A, H5N1, was identified in Hong Kong. The strain was rife in chickens and a few hundred people had become infected. Mortality in these individuals was very high, (6 of 18 died), and so there was serious concern that it marked the beginning of a new pandemic. The authorities in Hong Kong responded by a mass cull of poultry in the region and about 1.5 million chickens were slaughtered. H5N1 did not spread easily from person to person and no further cases were reported in people following the slaughter.
Whether the H5N1 outbreak was an isolated incident of a strain spreading from chicken to humans, or whether it was the start of a major pandemic which was nipped in the bud, cannot be known. Subsequent analysis showed that the high virulence of the new strain could partly be related to the new variant of haemagglutinin (H5), and partly to a more efficient viral polymerase. This outbreak clearly demonstrates the way in which bird influenza can act as a source of new viral strains, and shows that such new strains may be very dangerous to humans.
Since the discovery of the influenza virus in the 1930s it has been possible to isolate and accurately identify each of the epidemic strains, but, as earlier strains of virus have now died out, it has been necessary to infer their identity by examining the antibodies in the serum of affected people.
Antibodies and the ability of the immune system to respond to a strain of flu are much more persistent than the virus itself. It is thus possible to analyse antibodies to determine which types of haemagglutinin and neuraminidase they recognise long after the virus itself has gone. One can then deduce which type of influenza virus that person contracted earlier in their life (as explained in Section 5.2).
3.1 Tracking the emergence of new strains
Influenza is one of several diseases monitored by the WHO Global Alert and Response (GAR) network (WHO, 2011a), comprising 110 ‘sentinel’ laboratories in 82 countries. The organisation’s surveillance and monitoring of the disease then forms part of their Global Influenza Programme (GIP), and they use data gathered from participating countries to:
- provide countries, areas and territories with information about influenza transmission in other parts of the world to allow national policy makers to better prepare for upcoming seasons
- provide data for decision making regarding recommendations for vaccination and treatment
- describe critical features of influenza epidemiology including risk groups, transmission characteristics, and impact
- monitor global trends in influenza transmission
- inform the selection of influenza strains for vaccine production (WHO, 2011b).
The influenza data from the sentinel laboratories is fed into a global surveillance programme, started by the WHO in 1996, called FluNet (WHO, 2011c), which is one of the tools that facilitates the actions described above.
Activity 1 Using FluNet
Use the link below to visit the WHO’s FluNet web page and locate and view the chart showing the global circulation of flu (in the section marked ‘View charts’) to find out which flu subtypes are currently the major ones in circulation in the human population.
Link to WHO FluNet web page
At the time of writing (2011), Influenza A (H5N1) and influenza B (unknown lineage) are the two main types globally. FluNet also breaks this information down into geographic areas and countries so, if you wish, you can see what subtypes are circulating where you live.
Typically a flu vaccine contains material from the main influenza A strains and an influenza B strain, so that an immune response is induced against the most likely infections. Usually the scientists predict correctly and immunised people are effectively protected against the current strains (>90% protection). However, the prediction is occasionally incorrect, or a new strain develops during the time that the vaccine is being manufactured. In this case the vaccine generally provides poor protection.
What can you deduce about immunity against flu infection from the observations on vaccination above?
The immune response is strain-specific. If you are immunised against the wrong strain of flu, then the response is much less effective and you are more likely to contract the disease.
3.2 Immune responses to influenza
The immune system uses different types of immune defence against different types of pathogen. The responses against flu are typical of those which are mounted against an acute viral infection, but different from the responses against infection by bacteria, worms, fungi or protist parasites.
When confronted with an acute viral infection, the immune system has two major challenges:
- The virus replicates very rapidly, killing the cells it infects. Since a specific immune response takes several days to develop, the body must limit the spread of the virus until the immune defences can come into play.
- Viruses replicate inside cells of the body, but they spread throughout the host in the blood and tissue fluids. Therefore, the immune defences must recognise infected cells (intracellular virus) and destroy them. But the immune system must also recognise and eradicate free virus in the tissue fluids (extracellular virus) in order to prevent the virus from infecting new cells.
The kinds of immune defence that the body deploys against flu are briefly considered in the next section.
3.2.1 Summary of the response
How does the body act quickly to limit viral spread?
When a virus infects a cell of the body, the molecular machinery for protein synthesis within the cell is usurped as the virus starts to produce its own nucleic acids and proteins. The cell detects the flu dsRNA and other viral molecules and releases interferons, which bind to receptors on neighbouring cells and cause them to synthesise antiviral proteins. If a virus infects such cells they resist viral replication, so fewer viruses are produced and viral spread is delayed.
Also, in the earliest stages of a virus infection the molecules on the cell surface change. Cells lose molecules that identify them as normal ‘self’ cells. At the same time they acquire new molecules encoded by the virus. A group of large, granular lymphocytes recognise these changes and are able to kill the infected cell. This function is called ‘natural killer’ cell action and the lymphocytes that carry it out are termed NK cells.
Non-adaptive and adaptive responses
The actions of both interferons and NK cells in combating infection by influenza occur early in an immune response, and are not specific for the flu virus. These defences occur in response to many different kinds of viral infection, and they are part of our natural, or non-adaptive, immune responses.
Note that immunologists use the term non-adaptive to indicate a type of response that does not improve or adapt with each subsequent infection. This is quite different to its use in evolutionary biology, where it means ‘not advantageous’. Such non-adaptive immune responses slow the spread of an infection so that specific, or adaptive, immune defences can come into play.
The key features of an adaptive immune response are specificity and memory. The immune response is specific to a particular pathogen, and the immune system appears to ‘remember’ the infection, so that if it occurs again the immune response is much more powerful and rapid. Because an immune response is highly specific to a particular pathogen it often means that a response against one strain of virus is ineffective against another – if a virus mutates then the lymphocytes that mediate adaptive immunity are unable to recognise the new strain.
There are two principal arms of the adaptive immune system, mediated by different populations of lymphocytes. One group, called T-lymphocytes, or T cells (which develop in the thymus gland, overlying the heart), recognises antigen fragments associated with cells of the body, including cells which have become infected. A set of cytotoxic T cells (Tc) specifically recognises cells which have become infected and will go on to kill them. In this sense they act in a similar way to NK cells. However they differ from NK cells in that Tc cells are specific for one antigen or infectious agent, whereas NK cells are non-specific.
The second group of lymphocytes are B cells (that differentiate in the bone marrow), which synthesise antibodies that recognise intact antigens, either in body fluids or on the surface of other cells. Activated B cells progress to produce a secreted form of their own surface antibody. Antibodies that recognise the free virus act to target it for uptake and destruction by phagocytic cells.
Therefore the T cells and NK cells deal with the intracellular phase of the viral infection, while the B cells and antibodies recognise and deal with the extracellular virus.
The two reactions described above are illustrated in Figure 8.
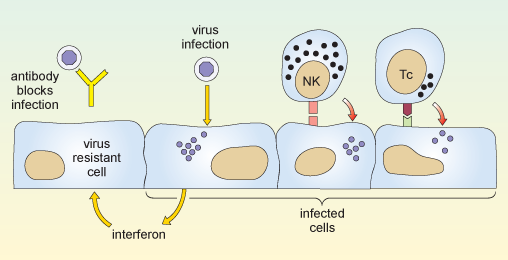
The first part of the diagram shows an antibody molecule binding to the virus and preventing it from entering the cell. The next part shows a virus entering and multiplying within a cell, causing the cell to release interferon. When the interferon reaches a neighbouring uninfected cell, it makes that cell resistant to infection. Also shown are a natural killer cell and a cytotoxic T cell. Either of these can bind to an infected cell via specific receptors on the cell surface, and kill that cell.
You might ask why it takes the adaptive immune response so long to get going. The answer is that the number of T cells and B cells that recognise any specific pathogen is relatively small, so first the lymphocytes which specifically recognise the virus must divide so that there are sufficient to mount an effective immune response. This mechanism is fundamental to all adaptive immune responses.
Activity 2 Influenza mini-lecture
Some of the themes that we have discussed up to now are presented in Video 1: a mini-lecture on influenza by David Male of The Open University. Watch the videos and then attempt the questions below
Transcript: Video 1 Influenza mini-lecture.
Influenza is a viral disease which generally starts with an infection of the upper respiratory tract and develops with systemic symptoms including fever and lassitude over the course of 1–2 weeks. Although it is debilitating, most people make a full recovery. However it may be fatal for older people and those with weaker immune systems, particularly if the virus disposes them to concurrent bacterial infections such as pneumonia.
The disease is caused by a group of myxoviruses, influenza A, B and C, although the most serious infections are caused by influenza A, and the following discussion refers to influenza A. The virus is seen in this transmission electron micrograph with proteins projecting from the viral envelope. Very many different variants of influenza have been identified since the virus was first discovered in 1935, and the variants are responsible for the different infections which occur in successive years. This is the reason that we can suffer from flu several times during our lives – in effect we become infected on each occasion with a different strain of virus, which our immune system has not encountered before and to which we are not immune.
Look at the overall structure of influenza A. The myxoviruses are all enveloped, that is to say that they have an outer membrane or envelope, which has been derived from the plasma membrane of an infected host cell. Two major viral proteins are present in the envelope, namely the haemagglutinin and the neuraminidase. Neuraminidase is an enzyme which cleaves sialic acid residues on glycoproteins and this protein performs an important function, in allowing the virus to bud off from the infected cell and spread through the body. The haemagglutinin is also essential for viral infection. It binds to carbohydrate groups present on glycophorins, molecules which occur on the surface of cells of the body. Binding of haemagglutinin to the surface of host cells is the first step in infection. Since the glycophorins are widely distributed on different cell types, influenza is able to cause widespread infection. As we shall see later, the antibody response against the haemagglutinin of the virus is critical in protecting us against on-going infection, although antibodies to the neuraminidase can also contribute to immunity. Within the viral envelope, the viral capsid is formed from the ‘M-protein’, which contains the virus’ genetic material, RNA, nucleoproteins, and a number of enzymes needed for replication.
The viral genome consists of 8 separate strands of RNA. Because the genome is fragmented in this way, it means that the different strains of virus can re-assort their genes relatively easily, and this is an important source of new viral strains.
Look now at the epidemic pattern of influenza over a number of years. The graph shows the number of isolates of different strains of influenza, in laboratories in the USA between 1995 and 1997. You can see that in temperate latitudes the infection rates follow a seasonal pattern, with more people developing the disease in the winter months. Examination of the structure of the haemagglutinin in successive years shows that minor mutations occur in the primary structure between the virus strains prevalent in each year. Although these do not affect the ability of the haemagglutinin to bind to host cells, the change is sufficient to prevent antibodies specific for a previous strain from binding to the new strain’s haemagglutinin. In effect, last year’s antibodies are unable to protect us from this year’s strain of flu.
The structure of the viral haemagglutinin can be seen in this model, which shows the backbone of the chain of amino acids. We will look at the way in which a neutralising antibody binds to an epitope on the haemagglutinin. The epitope is formed by amino acid residues in the loops at the exposed part of the molecule. Three loops which contribute residues to the epitope are highlighted here by space-filling the residues. Two domains of the heavy chain of the neutralising antibody are shown in yellow. The constant and variable domains are clearly visible, and the three hypervariable loops which contribute to the binding site are picked out in colour. You can see how the epitope and the heavy chain are complementary in shape. To complete the picture, we will add the light chain to the model. In this case, it is clear that the light chain contributes very little to the antibody combining site. When the space-filling model is completed, you can see that the residues forming the epitope are buried in the centre of the binding site and it is precisely these residues which are most likely to mutate between one viral strain and another. This progressive but limited change in the structure of the haemagglutinin is called genetic drift. Occasionally, perhaps once every 10–20 years, a major new strain of influenza appears which is radically different from those of previous years. Such a change is called genetic shift. The appearance of such new strains is associated with a worldwide serious epidemic of flu, called a pandemic. For example the pandemic strain of ‘Spanish flu’ which developed in 1918, had a different haemagglutinin and neuraminidase from the previously dominant strain, and this outbreak is thought to have caused the deaths of 20 million people world-wide. The picture shows a ward at the time. This epidemic particularly affected young fit people. One doctor wrote ‘it is only a matter of hours until death comes. It is horrible.’
The origin of new pandemic strains has been much debated, but it appears most likely that a human strain of flu exchanges genetic material with an animal strain of flu for example from ducks, or pigs. Such a reassortment of genes could occur if two different flu viruses simultaneously infect the same cell, and produce new viruses containing some gene segments from each type – remember that the flu virus has a segmented genome which allows this to occur.
The major pandemic strains are distinguished according to which haemagglutinin they have and which neuraminidase. So, for example in 1957 the dominant strain H1, N1 changed both its haemagglutinin and neuraminidase, and the new dominant strain H2N2 persisted until 1968. At present, (that is, in 2001) there are two dominant strains of influenza A in circulation H1N1 and H3N2.
One of the problems of producing vaccine for flu is that we do not know what next year’s major strain will be, and there is only a limited amount of time available before an epidemic spreads. The map shows the way in which the epidemic of Asian flu spread in 1957 from its origin in China in February, through South-East Asia by April and from there to all parts of the world by the end of the year. The figures on the map indicate the months in which the virus was isolated in different areas.
Nowadays, laboratories throughout the world track the appearance of new variants, and aim to identify the current circulating strains and any potentially new pandemic strains. Having decided the composition of the vaccine, there are just a few months to prepare it for the next flu season. Have a look at the current vaccine, it contains examples of the two major strains of influenza A H1N1 and H3N2 which are circulating and vaccine for the main current strain of influenza B. As there is insufficient time and resource to produce vaccine for everyone, the vaccine is usually recommended for older people and high risk groups, such as health professionals.
In addition to the antibody response, cytotoxic T cells are important in clearing virally-infected cells. The cytotoxic T cells recognise peptide fragments of several of the viral proteins, including the internal proteins such as the M-protein nucleoproteins and polymerases which are genetically stable. The bar chart shows the ability of lymphocytes from a single donor to kill cells which have been transfected with a single flu antigen. This is a measure of the prevalence of cytotoxic T cells for each of the proteins. Most of the cytotoxicity is directed against internal proteins shown on the green bars. Clones of cytotoxic cells against internal proteins usually recognise several of the major strains of flu. In contrast, those which recognise the external proteins are often, but not always strain-specific.
Even when a cytotoxic T cell recognises the same antigen as an antibody, it usually recognises a different portion. For example cytotoxic T cells specific for the haemagglutinin often recognise internal fragments of the antigen rather than the external epitopes recognised by antibodies. Moreover, since cytotoxic T cells recognise antigen presented by MHC molecules, and since MHC molecules are different in each individual, the T cells in each individual recognise different parts of the antigen. These bar charts show the response of two individuals against peptides from the haemagglutinin. T cells from the first person respond to four different regions of the molecule, with highly antigenic peptides centred on residues 100, 180, 300 and 400. T cells from the second individual recognise different regions of the haemagglutinin.
There is some evidence that individuals with specific MHC haplotypes may be more efficient than others at recognising and destroying influenza-infected cells.
In summary, influenza A gives us an example of extreme genetic variability, where successive dominant strains of flu emerge. The new strains are not susceptible to control by antibodies in the host population, and so individuals may suffer from repeated infections. Indeed the general immunity in the host population provides the selective pressure for the emergence of the new strains.
- Draw a labelled diagram of the structure of influenza A.
- How do pandemic strains of influenza A come about?
Your diagram should look like Figure 4 .
Pandemic strains of influenza A normally arise by simultaneous infection of a non-human host (typically poultry or pigs) with two or more strains of influenza A. Reassortment of the eight viral segments from each virus allows the generation of a new hybrid virus type, with a completely novel surface structure that has never been seen before by a host immune system (antigenic shift).
4 Antiviral treatments
Two classes of antiviral drugs are used to combat influenza: neuraminidase inhibitors and M2 protein inhibitors.
Why are antibiotics not used to combat influenza?
Influenza is a virus. Antibiotics only work against bacteria.
Neuraminidase inhibitors
Recall from Section 2.1 that neuraminidase is an enzyme that is present on the virus envelope and cleaves sialic acid groups found in the polysaccharide coating of many cells (especially the mucus coating of the respiratory tract). Neuraminidase is used to clear a path for the virus to a host cell and facilitates the shedding of virions from an infected cell. Inhibition of neuraminidase therefore helps prevent the spread of virus within a host and its shedding to infect other hosts.
The two main neuraminidase inhibitors currently in clinical use are zanamivir (trade name Relenza) and oseltamivir (trade name Tamiflu). These are effective against influenza A and B, but not influenza C which exhibits a different type of neuraminidase activity that only cleaves 9-O-acetylated sialic acid.
M2 inhibitors
Recall from Table 1 that the influenza M2 protein forms a pore that allows protons into the capsid, acidifying the interior and facilitating uncoating.
Drugs such as amantadine (trade name Symmetrel) and rimantadine (trade name Flumadine) block this pore, preventing uncoating and infection. However, their indiscriminate use in ‘over-the-counter’ cold remedies and farmed poultry has allowed many strains of influenza to develop resistance. Influenza B has a different type of M2 protein which is largely unaffected by these drugs.
5 Diagnosis of influenza
Many diseases produce symptoms similar to those of influenza; in fact, ‘flu-like’ is a term that is frequently used to describe several different illnesses. Since influenza spreads rapidly by airborne transmission and is a life-threatening condition in certain vulnerable groups, it is important that cases of the disease are identified as quickly as possible, so that preventative measures may be taken.
Most viral infections are not treated, although antiviral drugs such as zanamivir are used for potentially life-threatening cases or where the risk of transmission is high (as occurs during a pandemic).
Which sites in the body should be sampled for diagnosis?
The influenza virus infects the respiratory tract and is spread by coughing and sneezing, so specimens should be taken from the nose, throat or trachea.
In practice, the best specimens are nasal aspirates or washes, but swabs of the nose or throat may be used if they are taken vigorously enough to obtain cells. Ideally, samples should be taken within three days of the onset of illness, and all specimens need to be preserved in a transport medium and kept chilled until they reach the clinical microbiology laboratory.
5.1 Initial identification of influenza infection
Oral swabs or nasal aspirates are initially screened for the presence of a variety of respiratory viruses. This is done by extracting RNA from the sample and subjecting it to a reverse-transcription polymerase chain reaction (RT-PCR) as described below:
- Initially the RNA sample is reverse transcribed into complementary DNA (cDNA), using a commercially-available reverse transcriptase enzyme.
- The cDNA is then used in a standard PCR reaction to detect and amplify a short sequence of nucleotides specific to the virus. Multiple DNA sequences, each specific for a different type of virus, can be amplified in the same reaction, provided that these sequences are of different lengths.
- Each of the different amplified sequences is separated from the others when the entire sample is subjected to gel electrophoresis (an analytical technique in which molecules of different sizes move at various rates through a gel support in an applied electric field, thus making it possible to identify specific molecules.)
The polymerase chain reaction (PCR) technique is illustrated in Video 2.
Transcript: Video 2 PCR technique.
Polymerase chain reaction, or PCR, uses repeated cycles of heating and cooling to make many copies of a specific region of DNA. First, the temperature is raised to near boiling, causing the double-stranded DNA to separate, or denature, into single strands. When the temperature is decreased, short DNA sequences known as primers bind, or anneal, to complementary matches on the target DNA sequence. The primers bracket the target sequence to be copied. At a slightly higher temperature, the enzyme Taq polymerase, shown here in blue, binds to the primed sequences and adds nucleotides to extend the second strand. This completes the first cycle.
In subsequent cycles, the process of denaturing, annealing and extending are repeated to make additional DNA copies.
After three cycles, the target sequence defined by the primers begins to accumulate.
After 30 cycles, as many as a billion copies of the target sequence are produced from a single starting molecule.
Typically, nucleotide sequences specific to five types of virus are searched for in each sample: influenza A, influenza B, respiratory syncytial virus (Baltimore group V, (–)ssRNA virus, and a major cause of respiratory illness in young children), adenoviruses and enteroviruses. Those samples that test positive for influenza in the RT-PCR reaction are inoculated into cells in culture. Sufficient virus for a limited number of tests can be produced from such cultures within 24 hours, but they are often maintained for up to a week.
5.2 Determining the subtype of influenza
Immunofluorescence.
Confirmation of a case of influenza is usually achieved by performing tests on some of the inoculated cultured cells using reference fluorescent-labelled antisera provided by the WHO. A reference antiserum is a sample serum known to contain antibodies specific for the molecule to be assayed (in this case, haemagglutinin or neuraminidase). These antisera are prepared using purified haemagglutinin and neuraminidase and are monospecific, each antibody reacting only with one epitope e.g. H1 or H3.
For the test, an antibody is added to a sample of inoculated cells. Following a wash step, if the antibody remains bound to the cells then they fluoresce under appropriate illumination, indicating the presence of viral antigen on the cell surface. This diagnostic technique can identify influenza virus on infected cells in as little as 15 minutes. A positive result not only confirms the RT-PCR data, but gives additional information on the subtype of the virus.
Further PCR analyses
Standardised RT-PCR protocols exist to look for the presence of different haemagglutinin and neuraminidase subtypes, chiefly H1, H3, H5, N1 and N2. (Poddar, 2002). If the PCR analysis indicates a dangerous strain of influenza A e.g. H5N1, then it is instantly sent to a WHO reference laboratory for further tests.
Haemagglutination assays
Influenza has haemagglutinins protruding from its viral envelope, which it uses to attach to host cells prior to entry. These substances form the basis of a haemagglutination assay, in which viral haemagglutinins bind and cross-link (agglutinate) red blood cells added to a test well, causing them to sink to the bottom of the solution as a mat of cells. If agglutination does not occur, then the red blood cells are instead free to roll down the curved sides of the tube to form a tight pellet.
A related test called a haemagglutination-inhibition assay (HAI), incorporates antibodies against different subtypes of viral haemagglutinin. The antibodies bind and mask the viral haemagglutinin, preventing it from attaching to and cross-linking red blood cells.
A HAI assay can be set up in one of two ways: either a known reference antibody is added to an unknown virus sample, or known reference viral haemagglutinin is added to a sample of patient serum containing antibodies against influenza. This second version of the HAI assay can therefore be used long after the infection has passed, when virions are no longer present.
Haemagglutination and HAI assays have the advantage that they are simple to perform and require relatively cheap equipment and reagents. However, they can be prone to false positive or false negative results, if the sample contains non-specific inhibitors of haemagglutination (preventing agglutination) or naturally occurring agglutinins of red blood cells (causing agglutination).
If a confirmed influenza A isolate reacts weakly or not at all in HAI then this indicates an unknown variant of influenza A and the sample is immediately sent to a WHO reference laboratory for further tests.
Neuraminidase inhibition assay
Typing influenza isolates in terms of their neuraminidase makes use of the enzyme activity of this glycoprotein. The neuraminidase inhibition assay is performed in two parts. The first part determines the amount of neuraminidase activity in a patient influenza sample, as outlined in Figure 9a. A substrate (called fetuin) that is rich in sialic acid residues is added to a sample of the influenza virus, and the viral neuraminidase enzyme cleaves the substrate to produce free sialic acid.
Addition of a substance that inactivates the neuraminidase stops the reaction, and a chromogen (a colourless compound that reacts to produce a coloured end-product) that turns pink in the presence of free sialic acid is added. The intensity of the pink colour is proportional to the amount of free sialic acid and can be measured using a spectrophotometer.
This assay of neuraminidase activity allows the appropriate amount of virus sample to be determined, and this quantity is then used in the second part of the assay. If too much or too little virus is used, the resulting changes, and therefore the neuraminidase, may be undetectable.
In the second part of the assay (Figure 9b), viral samples from the patient are incubated with anti-neuraminidase reference antisera. Each of the reference antisera used for this test has antibodies that bind one particular neuraminidase variant, e.g. N1 or N2.
How can these antisera be used to type the neuraminidase variant?
If the antibodies bind the neuraminidase in the patient’s sample they inhibit its activity. This means that the patient’s neuraminidase cannot cleave the sialic acid from its test substrate fetuin, so no colour change will occur when the chromogen is added. Conversely, if the antibodies in the reference antiserum do not bind the neuraminidase in the patient’s sample, then the enzyme will remain uninhibited and the pink colour will be produced as before.
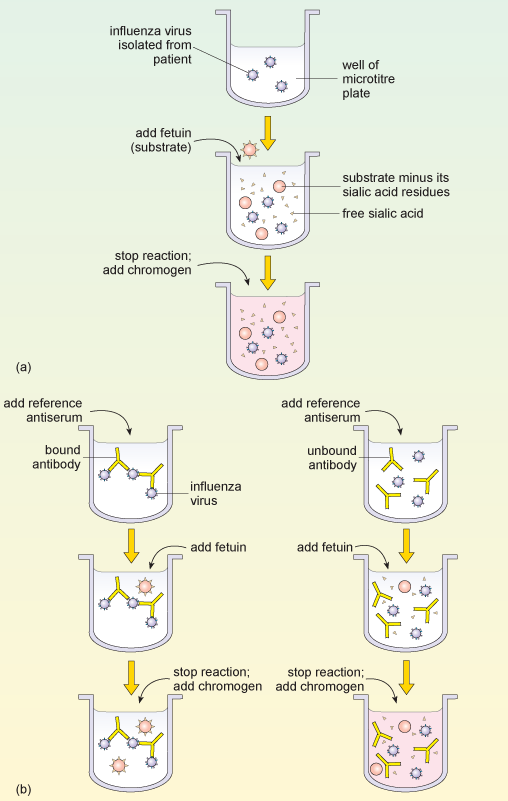
Part (a) of the diagram shows the sequence of obtaining a positive reaction in the neuraminidase inhibition assay. A patient sample containing influenza virus is added to the reaction well of a microtitre plate. Fetuin substrate is added, which is cleaved by viral neuraminidase into free sialic acid. A reagent is added that changes colour, dependent upon the amount of sialic acid present in the solution. Part (b) of the diagram shows how two different antibodies against neuraminidase can give two possible test outcomes for the patient sample from part (a). The diagram on the left shows what happens when the antibody binds to the neuraminidase and inactivates it (left side), while the right side diagram shows what happens when the antibody does not bind that type of neuraminidase. Fetuin and then the colour-change reagent are then added to both test wells. When the antibody fails to recognise the neuraminidase (right side) fetuin is cleaved by neuraminidase and gives a colour reaction. When the antibody recognises the neuraminidase (left side) its activity is blocked, fetuin is not cleaved and no colour reaction occurs.
6 Conclusion
As you reach the end of this free course you should consider some of the important points that the study of flu raises.
- A single pathogen can produce different types of disease in different people. Genetic variation in a pathogen can also affect the type of disease it produces. To understand this we need to know something of the genetic and social differences in the host population, and of the diversity of the pathogen.
- The symptoms of a particular disease may be produced by different pathogens or by a combination of pathogens. To understand this requires some knowledge of pathology and cell biology.
- Some diseases, such as flu, affect humans and several other animal species, whereas others are more selective in their host range. The basic biology of different pathogens underlies these differences.
- Flu is a disease that can be contracted several times during a lifetime, but many other infectious diseases are only ever contracted once. To understand this we need to look at how the immune system reacts to different pathogens, and how responses vary depending on the pathogen.
- Outbreaks of flu occur regularly, but some epidemics are much more serious than others. This requires an understanding of aspects of virology, immunology, evolutionary biology and epidemiology.
7 Questions for the course
The following questions allow you to assess your understanding of the content of this course. Each one relates to one or more of the intended learning outcomes of the study.
If you are unable to answer a question, or do not understand the answer given, then reread the relevant section(s) of the course and try the question again.
(This question relates to case study learning outcome (LO) 2.)
Why would Robert Koch have been unable to demonstrate that influenza viruses cause the disease influenza, according to his own postulates?
Koch’s second postulate states that the pathogen can be isolated in pure culture on artificial media. Viruses can only multiply within host cells, so Koch would have been unable to isolate the virus using artificial media. (Much later, eggs and live cells in tissue culture came to be used for growing flu virus, but this was long after Koch’s death, and they do not strictly conform to the original postulate.)
(This question relates to LO2.)
List the various structural components of an influenza A virus and note where each of these elements is synthesised within an infected cell.
Viral RNA is synthesised in the nucleus of the infected cell. The M-protein and other internal proteins are synthesised on ribosomes in the cytoplasm. The capsid is then assembled in the nucleus. The haemagglutinin and neuraminidase are synthesised on ribosomes on the endoplasmic reticulum. The envelope is derived from the host cell’s own plasma membrane.
(This question relates to LO2 and LO4.)
It is very uncommon for a strain of influenza that infects other animals to infect people; nevertheless such strains are very important for human disease. Why is this?
Animal strains of influenza act as a reservoir of genes that may recombine with human influenza viruses to produce new strains that can spread rapidly in humans. Such pandemic strains frequently produce serious diseases with high mortality.
(This question relates to LO2 and LO3.)
Which immune defences are able to recognise and destroy virally-infected host cells?
Cytotoxic T cells and NK cells are able to recognise and destroy virally-infected host cells.
(This question relates to LO4 and LO5.)
Why do most people suffer from influenza several times in their lives?
The virus mutates regularly (antigenic drift); also new strains are occasionally generated by recombination (antigenic shift). Since the immune response is generally specific for a particular strain of virus, new strains are not susceptible to immune defences which have developed against earlier strains.
Further reading
Acknowledgements.
This course was written by Jon Golding and Hilary MacQueen.
Except for third party materials and otherwise stated (see terms and conditions ), this content is made available under a Creative Commons Attribution-NonCommercial-ShareAlike 4.0 Licence .
Course image: thierry ehrmann in Flickr made available under Creative Commons Attribution 2.0 Licence .
The material acknowledged below is Proprietary and used under licence (not subject to Creative Commons Licence). Grateful acknowledgement is made to the following sources for permission to reproduce material in this course:
Figure 1: Prescott, L., Harley, J., and Klein, D. (1999) Microbiology, 4th ed. Copyright © The McGraw-Hill Companies
Figure 3: Noymer, A., and Garenne, M. (2000) ‘The 1918 influenza epidemic’s effects on sex differentials in mortality in the United States’, Population and Development Review , Vol 26 (3) 2000, The Population Council
Figure 6: CNRI/Science Photo Library;
Figure 7: Bammer, T. L. et al. (April 28, 2000) ‘Influenza virus isolates’ reported from WHO, Surveillance for Influenza – United States , Centers for Disease Control and Prevention
Video 1: Immunology Interactive (Male, Brostoff and Roitt) copyright the authors, reproduced by permission of David Male
Video 2: with kind permission from the Howard Hughes Medical Institute.
Every effort has been made to contact copyright owners. If any have been inadvertently overlooked, the publishers will be pleased to make the necessary arrangements at the first opportunity.
Don't miss out:
If reading this text has inspired you to learn more, you may be interested in joining the millions of people who discover our free learning resources and qualifications by visiting The Open University - www.open.edu/ openlearn/ free-courses
Copyright © 2019 The Open University
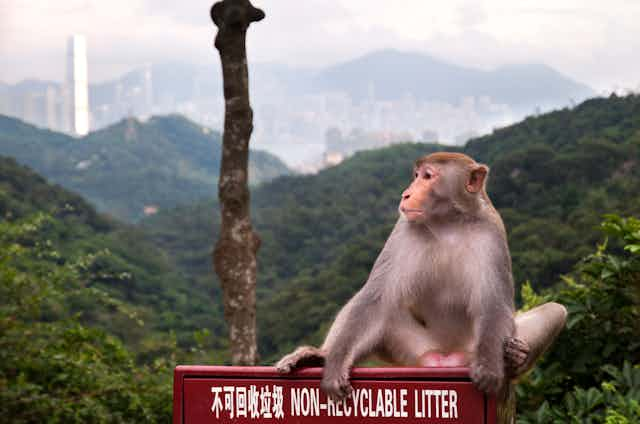
Man who caught virus from a monkey in a critical condition – B virus explained
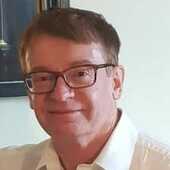
Deputy Lead, School of Medicine, University of Central Lancashire
Disclosure statement
Colin Michie does not work for, consult, own shares in or receive funding from any company or organisation that would benefit from this article, and has disclosed no relevant affiliations beyond their academic appointment.
University of Central Lancashire provides funding as a member of The Conversation UK.
View all partners
A 37-year-old man wounded by a wild monkey in Hong Kong is in intensive care suffering from infection with B virus.
Although this is the first reported case of a B virus infection in a human in Hong Kong, it is not the first in the world. The US Centers for Disease Control and Prevention has recorded 50 cases of human infection since 1932, when the virus was first identified. Twenty-one of those infected died .
The first person – as far as we know – to catch B virus was a young doctor called William Brebner . He was bitten by a macaque monkey while researching poliovirus. The bite wound healed, but neurological illness eventually ensued, leading to respiratory failure. Brebner died at Bellevue Hospital, New York, in November 1932.
After his death, his brain tissues were found to cause neurological damage when injected into rabbits. Brebner’s colleagues Albert Sabin and Arthur Wright labelled this agent “B” .
We now know that the virus belongs to the herpes family, hence its other moniker: “herpes virus B”. It is a biosafety category 4 virus , the highest level – the same as Ebola and unknown new pathogens.
Prompt treatment with antiviral drugs can be lifesaving, but B virus remains a deadly pathogen. If not treated promptly, it has a mortality rate of 70% . There is no vaccine against this virus.
Most cases of B virus infection have involved laboratory workers handling captive animals or monkey tissues, rather than those living alongside these excitable, toothy animals in the wild.
Old World primates
In this most recent case, the 37-year-old man was attacked by macaques at Hong Kong’s Kam Shan Country Park – known locally as Monkey Hill. There are about 1,800 wild monkeys in Hong Kong, including two different macaque species. The authorities are warning people not to approach these creatures.
Macaques are a particularly adaptable group of old world primates with a wide global distribution. They live in towns, villages and temples across Asia and have been introduced elsewhere. They include the Barbary apes of Gibraltar, which were provided with rations by the British army after the second world war.
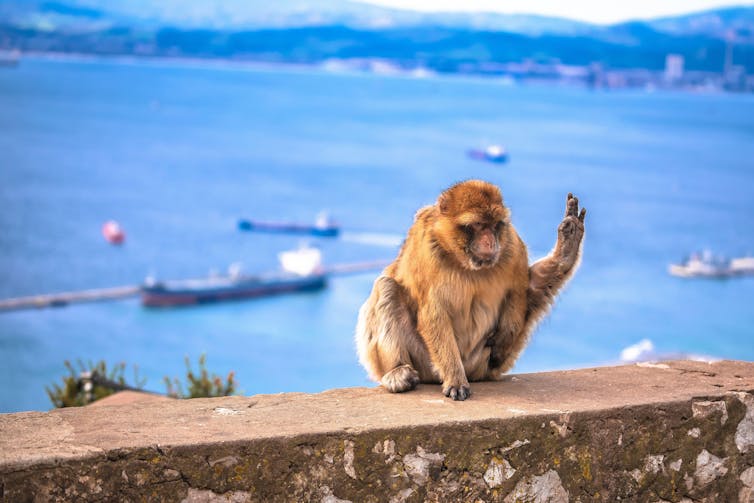
They are eaten as a delicacy in Indonesia and sold elsewhere as bush meat. Indonesian folklore through the centuries provides detailed observations of these creatures, but reassuringly no evidence that macaques caused harm to humans.
Despite lots of contact with many humans, there are remarkably few recorded episodes of spill-over of B virus outside laboratories. The virus does not spread easily .
A few cases show that bites and scratches from a macaque, or contact with the monkey’s mouth, nose or body fluids can transfer B virus that may be on the animal’s skin or in its mouth. (Most macaque populations harbour B virus, but without symptoms.)
Signs of infection in a human usually develop within two to 30 days. There may be blisters around the contact point or wound, together with a flu-like illness.
The virus can spread to lymph nodes and the nervous system. This causes agitation, confusion, double vision, trouble with coordination and muscle weakness.
Patients can develop hiccups if this inflammation involves the brain stem. If it reaches the lungs, they may need to be admitted to an intensive care unit.
No immediate fear
Spread of this virus between humans is highly unlikely. Only one account describes the spread of B virus from one human to another. The contact case was linked to the use of a cream previously used on a patient .
There is no evidence of a new B virus strain in the primates on Monkey Hill, so this case of herpes B has no features of a new zoonosis. However, it should raise our awareness of the potential risks posed by viruses carried by animals.
- Zoonotic diseases
- Laboratory animals

Program Manager, Teaching & Learning Initiatives

Lecturer/Senior Lecturer, Earth System Science (School of Science)

Sydney Horizon Educators (Identified)

Deputy Social Media Producer

Associate Professor, Occupational Therapy
Learn how UpToDate can help you.
Select the option that best describes you
- Medical Professional
- Resident, Fellow, or Student
- Hospital or Institution
- Group Practice
- Patient or Caregiver
- Find in topic
RELATED TOPICS
INTRODUCTION
The following topic will outline issues related to the management of hepatitis B through the use of cases studies that incorporate patient-specific clinical information and test results. Our approach to treatment is generally consistent with guidelines from the European Association for the Study of the Liver guidelines, Asian-Pacific Association for the Study of the Liver guidelines, and American Association for the Study of Liver Diseases Practice Guidelines and Guidance [ 1-5 ].
Additional topic reviews that address the diagnosis and management of HBV include:
● (See "Hepatitis B and pregnancy" .)
● (See "Clinical manifestations and diagnosis of hepatitis B virus infection in children and adolescents" and "Management of hepatitis B virus infection in children and adolescents" .)
Thank you for visiting nature.com. You are using a browser version with limited support for CSS. To obtain the best experience, we recommend you use a more up to date browser (or turn off compatibility mode in Internet Explorer). In the meantime, to ensure continued support, we are displaying the site without styles and JavaScript.
- View all journals
- Explore content
- About the journal
- Publish with us
- Sign up for alerts
Latest science news, discoveries and analysis
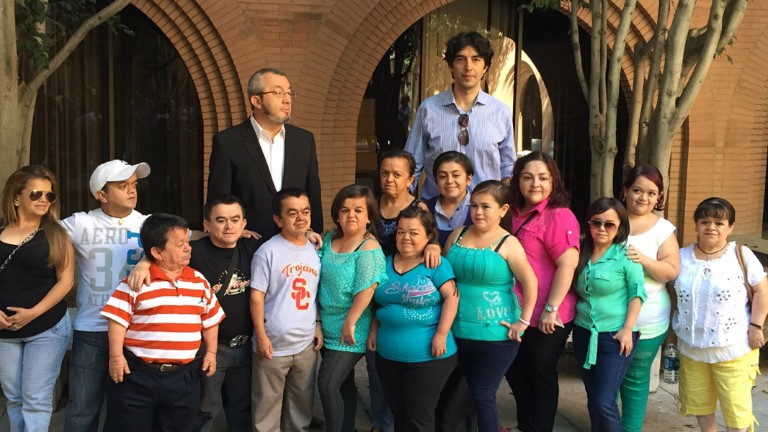
Could a rare mutation that causes dwarfism also slow ageing?
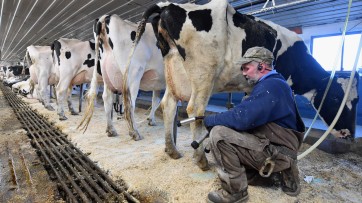
Bird flu in US cows: is the milk supply safe?
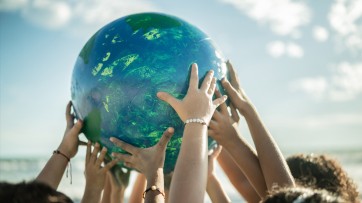
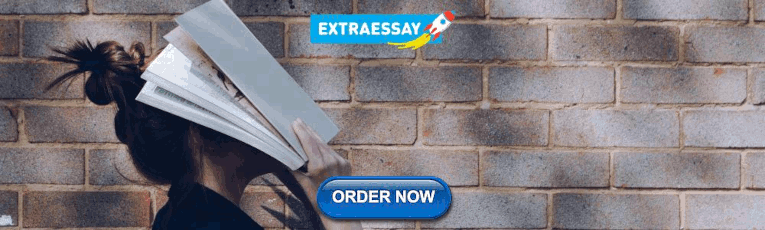
Future of Humanity Institute shuts: what's next for ‘deep future’ research?
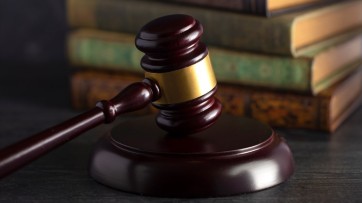
Judge dismisses superconductivity physicist’s lawsuit against university
Nih pay raise for postdocs and phd students could have us ripple effect, hello puffins, goodbye belugas: changing arctic fjord hints at our climate future, china's moon atlas is the most detailed ever made, ‘shut up and calculate’: how einstein lost the battle to explain quantum reality, ecologists: don’t lose touch with the joy of fieldwork chris mantegna.
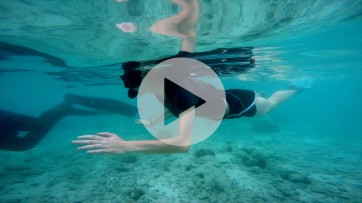
Should the Maldives be creating new land?
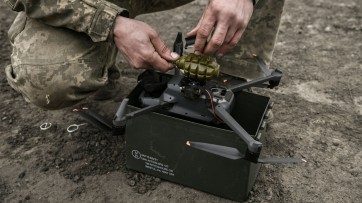
Lethal AI weapons are here: how can we control them?
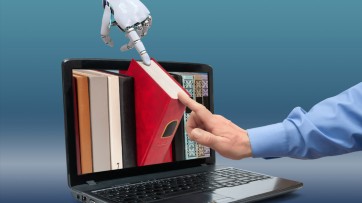
Algorithm ranks peer reviewers by reputation — but critics warn of bias
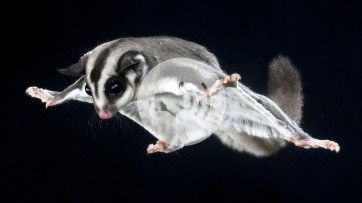
How gliding marsupials got their ‘wings’
Bird flu virus has been spreading in us cows for months, rna reveals, audio long read: why loneliness is bad for your health, nato is boosting ai and climate research as scientific diplomacy remains on ice, rat neurons repair mouse brains — and restore sense of smell.
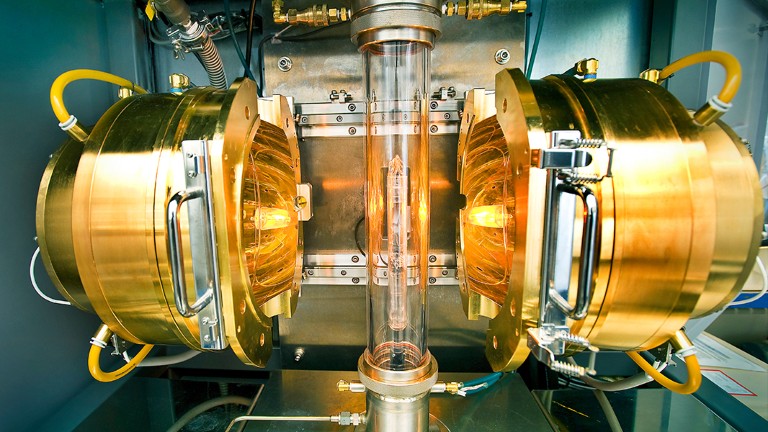
Retractions are part of science, but misconduct isn’t — lessons from a superconductivity lab
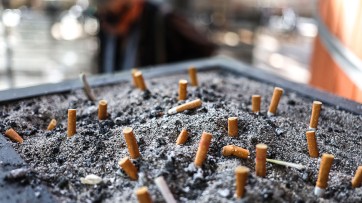
Any plan to make smoking obsolete is the right step
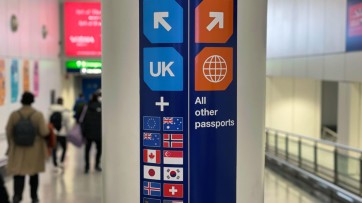
Citizenship privilege harms science
European ruling linking climate change to human rights could be a game changer — here’s how charlotte e. blattner, will ai accelerate or delay the race to net-zero emissions, current issue.
The Maldives is racing to create new land. Why are so many people concerned?
Surprise hybrid origins of a butterfly species, stripped-envelope supernova light curves argue for central engine activity, optical clocks at sea, research analysis.
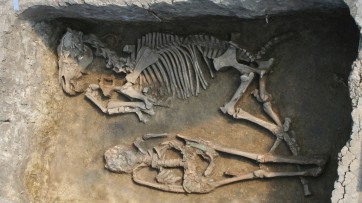
Ancient DNA traces family lines and political shifts in the Avar empire
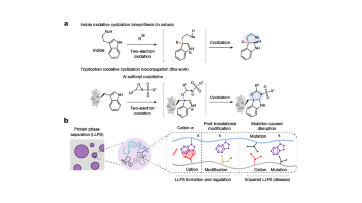
A chemical method for selective labelling of the key amino acid tryptophan
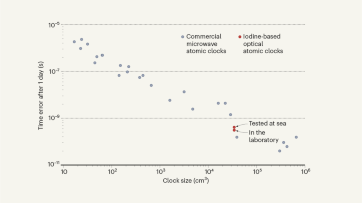
Robust optical clocks promise stable timing in a portable package
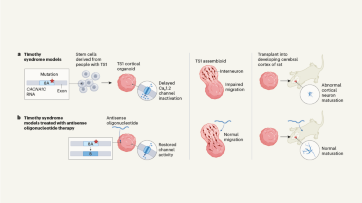
Targeting RNA opens therapeutic avenues for Timothy syndrome
Bioengineered ‘mini-colons’ shed light on cancer progression, galaxy found napping in the primordial universe, tumours form without genetic mutations, marsupial genomes reveal how a skin membrane for gliding evolved.
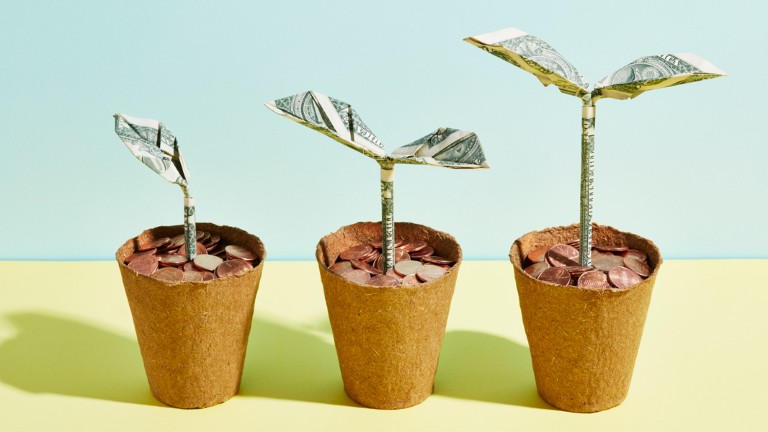
Scientists urged to collect royalties from the ‘magic money tree’
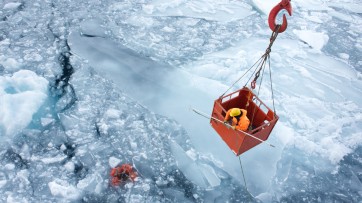
Breaking ice, and helicopter drops: winning photos of working scientists
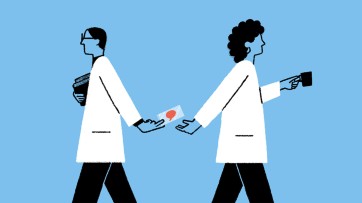
Shrouded in secrecy: how science is harmed by the bullying and harassment rumour mill
Want to make a difference try working at an environmental non-profit organization, how ground glass might save crops from drought on a caribbean island, books & culture.
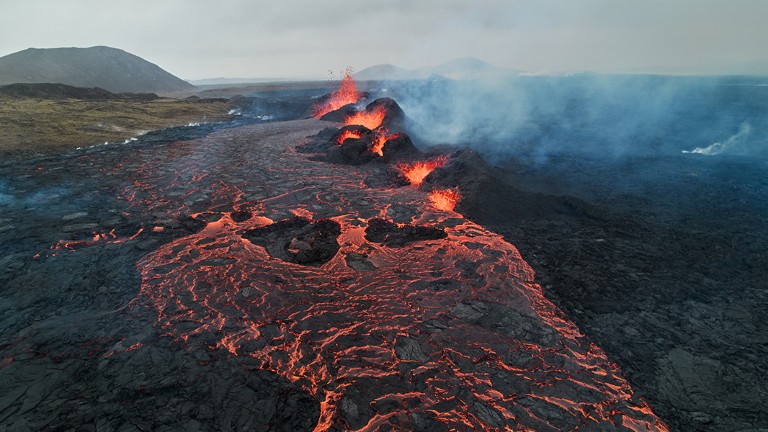
How volcanoes shaped our planet — and why we need to be ready for the next big eruption
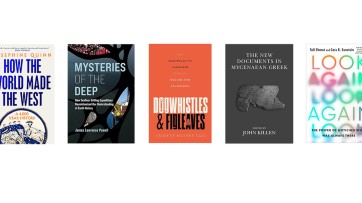
Dogwhistles, drilling and the roots of Western civilization: Books in brief
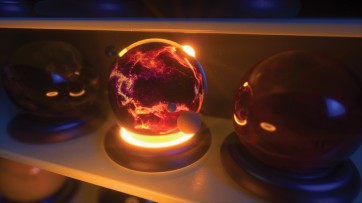
Cosmic rentals
Las borinqueñas remembers the forgotten puerto rican women who tested the first pill, dad always mows on summer saturday mornings, nature podcast.
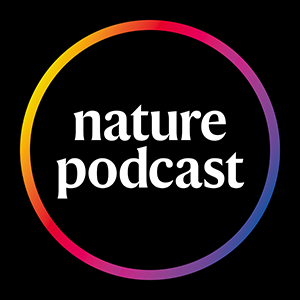
Latest videos
Nature briefing.
An essential round-up of science news, opinion and analysis, delivered to your inbox every weekday.

Quick links
- Explore articles by subject
- Guide to authors
- Editorial policies
Avian Influenza A(H5N1) U.S. Situation Update and CDC Activities
Current Situation Highlights Importance of Preventive Measures for People with Exposures
April 19, 2024 – CDC continues to respond to the public health challenge posed by the outbreak of avian influenza A(H5N1) virus, or “H5N1 bird flu” in dairy cows and other animals in the U.S. CDC is collaborating with partners including the U.S. Department of Agriculture (USDA), the Food and Drug Administration (FDA), and state public health and animal health officials to address this emerging infectious disease using a One Health approach . USDA is now reporting that eight U.S. states have outbreaks in dairy cattle and that the virus has spread through cattle movement between herds and also from dairy cattle premises into nearby poultry premises and has infected a number of barn cats. However, only one associated human case to date has been linked with this outbreak in dairy cows and was reported by Texas on April 1, 2024. [1] CDC’s response to this unprecedented outbreak of influenza A(H5N1) in dairy cattle and other animals most recently includes:
- Supporting states monitoring people with exposure to cows, birds, or other domestic or wild animals infected or potentially infected with influenza A(H5N1) viruses. Testing for these people is being done by state or local officials using a CDC test, and CDC is conducting confirmatory testing when needed.
- Continuing to work in the laboratory to better characterize the virus from the human case in Texas. This week CDC completed susceptibility testing for influenza antiviral medications that are used for seasonal influenza (e.g., the neuraminidase inhibitors oseltamivir, zanamivir and peramivir). Testing confirmed that the A(H5N1) virus was susceptible to all commercially available FDA-approved and recommended neuraminidase inhibitor antivirals. Testing to confirm susceptibility to baloxavir marboxil, a different antiviral medication, takes longer and is ongoing.
- Studying human sera (blood) from people vaccinated against A(H5) to confirm that existing A(H5N1) candidate vaccine viruses (CVVs) will provide protection against the A(H5N1) virus isolated from the human case in Texas. Manufacturers could use these CVVs to make a vaccine if needed. Preliminary genetic analysis had suggested two existing CDC CVVs would offer protection against the virus isolated from the human case in Texas.
- Designing an epidemiological field study and preparing a multilingual and multidisciplinary team to travel on site to better understand the current outbreak, particularly the public health and One Health implications of the emergence of this virus in cattle.
- Engaging One Health partner organizations from public health, agriculture, milk regulatory officials, and others to share information and ensure preparedness to prevent and respond to this emerging infectious disease threat and for any potential human infections.
- Monitoring emergency department data and flu testing data in areas where A(H5N1) bird flu viruses have been detected in dairy cattle or other animals for any unusual trends in flu-like illness, flu, or conjunctivitis. So far, these data remain in expected ranges, and to date, surveillance systems do not show any unusual trends or activity.
This is a rapidly changing, emerging situation and CDC is committed to providing frequent and timely updates.
What Might Happen
The wide geographic spread of A(H5N1) bird flu viruses in wild birds, poultry, and some other mammals, including in cows, is creating additional opportunities for people to be exposed to these viruses. Therefore, there could be an increase in sporadic human infections resulting from bird, cattle, and other animal exposures, even if the risk of these viruses spreading to people has not increased. Sporadic human infections in the current context would not significantly change CDC’s risk assessment.
What Would Increase Public Health Risk
Identification of multiple simultaneous instances of A(H5N1) bird flu viruses spreading from birds, cattle, or other animals to people or certain genetic changes in circulating viruses could change CDC’s risk assessment because they could indicate the virus is adapting to spread more easily from animals to people. Additionally, if limited, non-sustained, person-to-person spread with this virus were to occur, that would also raise the public health threat because it could mean the virus is adapting to spread between people. Sustained person-to-person spread is needed for a pandemic to occur.
Because of the potential for influenza viruses to constantly change, continued surveillance and preparedness efforts are critical, and CDC is taking measures to be ready in case the current risk assessment for the general public changes. The immediate goal is to prevent further spread of this virus between animals and people. CDC will continue to monitor these viruses and update and adjust guidance as needed. As a reminder, while CDC believes the current risk of A(H5N1) infection to the general public remains low, people with close, prolonged, or unprotected exposures to infected birds, cattle, or other animals, or to environments contaminated by infected birds, cattle, or other animals, are at a greater risk of infection. CDC has interim recommendations for prevention, monitoring, and public health investigations of A(H5N1) viruses. CDC also has recommendations for worker protection and use of personal protective equipment (PPE) to reduce the risk of exposure . Compliance with these recommendations is central to containing the public health risk. Additionally, unpasteurized (“raw”) milk from sick cattle has tested positive for A(H5N1) viruses. Consumption of raw milk can be dangerous and is not recommended. The FDA has Questions and Answers Regarding Milk Safety During Highly Pathogenic Avian Influenza (HPAI) Outbreaks | FDA .
[1] The first human case of A(H5N1) bird flu in the United States was reported in 2022 in a person in Colorado who had direct exposure to poultry and was involved in the depopulating of poultry with presumptive A(H5N1) bird flu. The person recovered. Learn more at U.S. Case of Human Avian Influenza A(H5) Virus Reported | CDC Online Newsroom | CDC.
Descargo de responsabilidad: Es posible que en este sitio encuentre algunos enlaces que le lleven a contenido disponible sólo en inglés. Además, el contenido que se ha traducido del inglés se actualiza a menudo , lo cual puede causar la aparición temporal de algunas partes en ese idioma hasta que se termine de traducir (generalmente en 24 horas). Llame al 1-800-CDC-INFO si tiene preguntas sobre la influenza estacional, cuyas respuestas no ha encontrado en este sitio. Agradecemos su paciencia.
To receive email updates about this page, enter your email address:
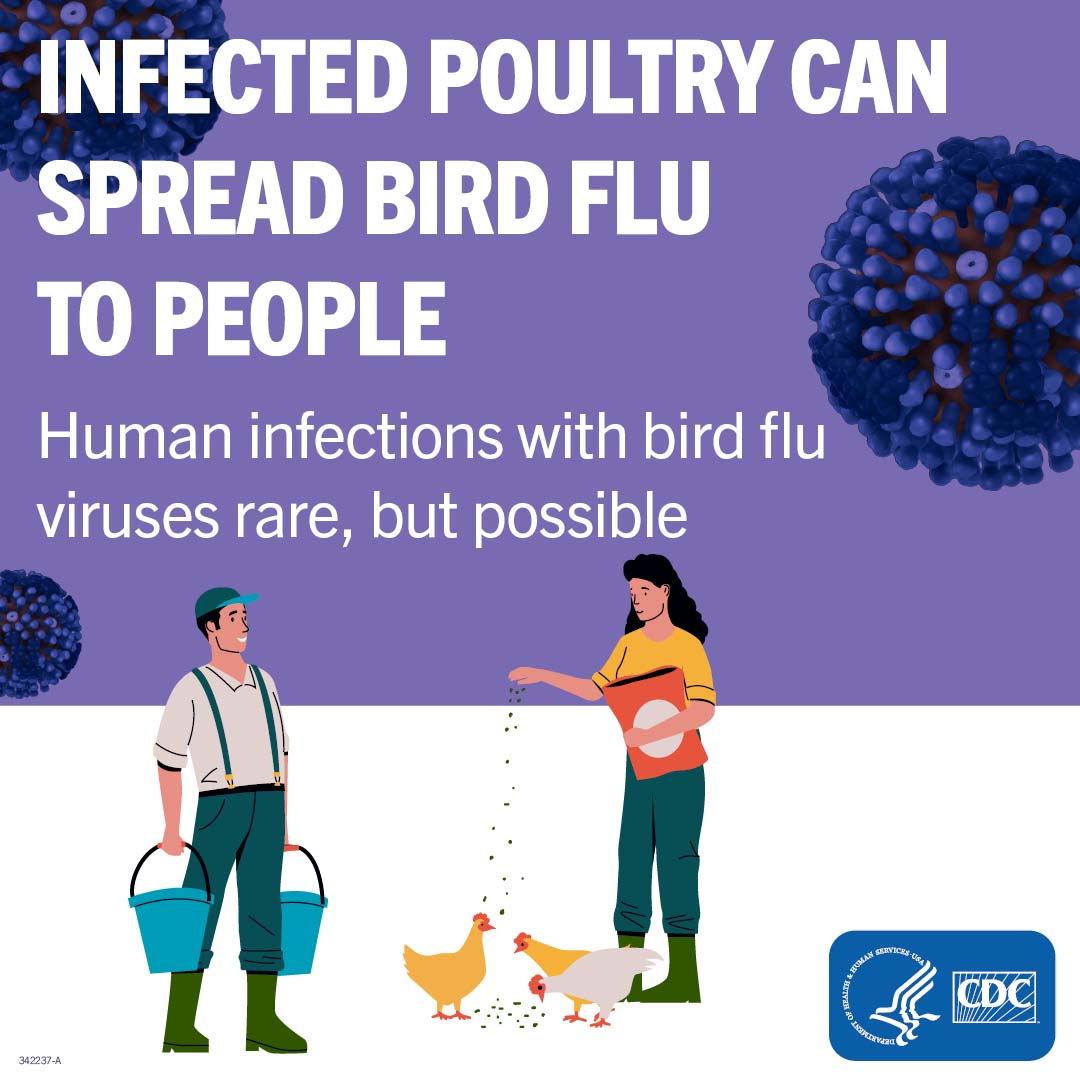
- Swine/Variant
- Influenza in Animals
Exit Notification / Disclaimer Policy
- The Centers for Disease Control and Prevention (CDC) cannot attest to the accuracy of a non-federal website.
- Linking to a non-federal website does not constitute an endorsement by CDC or any of its employees of the sponsors or the information and products presented on the website.
- You will be subject to the destination website's privacy policy when you follow the link.
- CDC is not responsible for Section 508 compliance (accessibility) on other federal or private website.
share this!
April 23, 2024
This article has been reviewed according to Science X's editorial process and policies . Editors have highlighted the following attributes while ensuring the content's credibility:
fact-checked
peer-reviewed publication
trusted source
Study sheds new light on cross-species virus spillovers that can cause pandemics
by University of Stirling
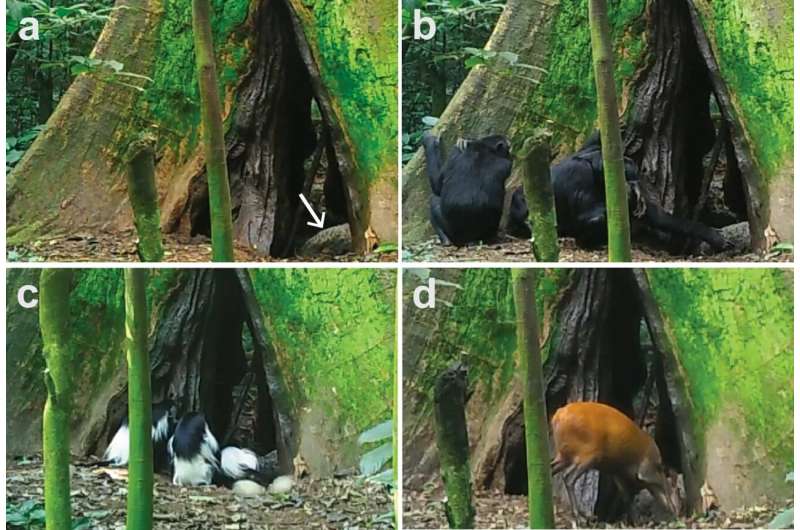
A study led by the University of Stirling jointly with the University of Wisconsin-Madison has shed new light on cross-species virus spillovers that can cause pandemics.
A researcher from the Faculty of Natural Sciences at Stirling discovered animals in an African forest eating bat excrement known as guano after a key food source in the region disappeared following selective deforestation. The paper "Selective deforestation and exposure of African wildlife to bat-borne viruses" was published in the journal Communications Biology .
Lab analysis of the bat guano identified a range of viruses, including a betacoronavirus related to SARS-CoV-2, the virus that caused the COVID-19 pandemic. It remains unknown whether the betacoronavirus found in the guano is transmissible to humans, but it does offer an example of how new infections might jump species barriers.
The study was prompted when Dr. Pawel Fedurek observed wild chimpanzees consume bat guano from a tree hollow in Budongo Forest, Uganda.
Dr. Fedurek then set up cameras which recorded chimps, monkeys and antelope eating the excrement.
The guano is an alternative source of crucial minerals after the palm trees the mammals once consumed were harvested to extinction locally. The palm was used by people in Budongo to dry tobacco leaves which are then sold to international companies.
Scientists do not yet fully understand the earliest stages of virus spillovers, which can lead to the deaths of tens of thousands of people, but they are thought to involve complex causal chains that begin with humans altering the environment.
Dr. Pawel Fedurek, an expert in animal behavior at the University of Stirling, said, "Our research illustrates how a subtle form of selective deforestation, ultimately driven by a global demand for tobacco, can expose wildlife and, by extension, humans to viruses residing in bat guano, increasing virus spillover risk.
"Studies like ours shed light on the triggers and pathways of both wildlife-to-wildlife and wildlife-to-human virus transmission, ultimately improving our abilities to prevent outbreaks and pandemics in the future.
"Most research into outbreaks and pandemics has focused on curtailing the spread of the virus, by finding an effective vaccine for example, rather than preventing animal-to-human virus transmission from happening in the first place. Our work stresses the importance of studying disease ecology before diseases enter humans."
After discovering mammals consuming guano, Dr. Fedurek and the project co-lead Dr. Caroline Asiimwe (then Conservation Coordinator of the Budongo Conservation Field Station, Uganda) involved Professor Tony Goldberg (University of Wisconsin-Madison, U.S.), who is a world-renowned expert in epidemiology and evolution of infectious diseases.
Professor Goldberg identified the viruses in the guano in collaboration with researchers who quantified the mineral content of the guano (Professor Jessica Rothman, Hunter College of the City University of New York, U.S.) and established the probability of the novel betacoronavirus to infect the three mammalian species and humans (Gregory K. Rice, Naval Medical Research Command, U.S.).
Due to the magnitude and complexity of the laboratory analyses involved, the entire project took around six years to complete.
Textbook example
Professor Goldberg said, "Our study links tobacco farming to exposure of at least three species of African wildlife to viruses of bats. We believe this is a textbook example of how new infections might jump species barriers even before they get into humans.
"This is important since the initial stages of the onset of outbreaks, epidemics and pandemics have been particularly elusive for science.
"We may have stumbled upon a series of events that is usually hidden from the view of epidemiologists and public health officials. Our study might be particularly relevant to the origins of bat-borne disease such as coronaviruses and, perhaps, Ebola."
It is hoped the discovery may make it possible to enact interventions that break these sorts of causal chains, ultimately helping prevent future pandemics.
Health consequences
Dr. Asiimwe said, "Our study demonstrates that human activities that alter the environment can lead to devastating health consequences to both wildlife and humans and thus we should urgently learn to use natural resources sustainably.
"Considerable research efforts are also needed to investigate how forest degradation, and other forms of human activity, can affect wildlife's behavior in a way that exposes them and humans to dangerous viruses."
Journal information: Communications Biology
Provided by University of Stirling
Explore further
Feedback to editors

Global study shows a third more insects come out after dark
16 hours ago

Cicada-palooza! Billions of bugs to blanket America
19 hours ago

Getting dynamic information from static snapshots

Ancient Maya blessed their ballcourts: Researchers find evidence of ceremonial offerings in Mexico

Optical barcodes expand range of high-resolution sensor
Apr 26, 2024

Ridesourcing platforms thrive on socio-economic inequality, say researchers

Did Vesuvius bury the home of the first Roman emperor?

Florida dolphin found with highly pathogenic avian flu: Report

A new way to study and help prevent landslides

New algorithm cuts through 'noisy' data to better predict tipping points
Relevant physicsforums posts, is 5 milliamps at 240 volts dangerous.
3 hours ago
The Cass Report (UK)
Apr 24, 2024
Major Evolution in Action
Apr 22, 2024
If theres a 15% probability each month of getting a woman pregnant...
Apr 19, 2024
Can four legged animals drink from beneath their feet?
Apr 15, 2024
Mold in Plastic Water Bottles? What does it eat?
Apr 14, 2024
More from Biology and Medical
Related Stories

Surveillance in Vietnam highlights hotspots for viral disease emergence
Apr 26, 2023

Virus ancestry could help predict next pandemic
Feb 5, 2024

Genome study shows humans pass more viruses to animals than we catch from them
Mar 25, 2024

COVID-19 poo test for bats may help pandemic monitoring and conservation efforts
May 19, 2022

Why are animal-to-human diseases on the rise?
Apr 7, 2023

Understanding the human-animal interplay of COVID-19 and other diseases
Mar 31, 2022
Recommended for you

AI deciphers new gene regulatory code in plants and makes accurate predictions for newly sequenced genomes

Experiment reveals strategic thinking in mice

Scientists suggest using mobile device location data for studying human-wildlife interactions
Let us know if there is a problem with our content.
Use this form if you have come across a typo, inaccuracy or would like to send an edit request for the content on this page. For general inquiries, please use our contact form . For general feedback, use the public comments section below (please adhere to guidelines ).
Please select the most appropriate category to facilitate processing of your request
Thank you for taking time to provide your feedback to the editors.
Your feedback is important to us. However, we do not guarantee individual replies due to the high volume of messages.
E-mail the story
Your email address is used only to let the recipient know who sent the email. Neither your address nor the recipient's address will be used for any other purpose. The information you enter will appear in your e-mail message and is not retained by Phys.org in any form.
Newsletter sign up
Get weekly and/or daily updates delivered to your inbox. You can unsubscribe at any time and we'll never share your details to third parties.
More information Privacy policy
Donate and enjoy an ad-free experience
We keep our content available to everyone. Consider supporting Science X's mission by getting a premium account.
E-mail newsletter
Study reveals promising results for abatacept for severe COVID
A new study shows good results in patients with severe COVID-19 for abatacept, an anti-inflammatory immunomodulating drug used to treat psoriatic arthritis, according to findings published yesterday in JAMA Network Open .
The study was based on results seen among 395 hospitalized patients in the ACTIV-1 IM randomized clinical trial, designed to assess the efficacy of abatacept, which inhibits T-cell activation, reducing multiple inflammatory cytokines. A previous ACTIV-1 trial showed the drug decreased mortality in hospitalized patients with COVID-19, but optimal dosing of the drug based on body weight is still unknown, the authors said.
"Because increased body weight is a risk factor for severe COVID-19 and is associated with increased abatacept clearance (CL), it is possible that the pharmacokinetics of abatacept may be different in hospitalized patients with COVID-19, " the authors said.
Increased doses linked to recovery
Abatacept was administered to enrolled participants on day 1 as a single 10-milligram (mg)-per-kilogram intravenous infusion, with a maximum dose of 1,000 mg. At least two blood samples were collected through day 28, with clinical outcomes also assessed over 4 weeks.
Over the course of the study, 320 patients (81.0%) recovered, 32 (8.1%) did not recover, and 43 (10.9%) died. Every 5,000-unit increase of abatacept was associated with a higher probability of recovery at day 28, with a hazard ratio of 2.63 (95% confidence interval [CI], 1.70 to 4.08).
Overall, participants with higher abatacept exposure had improved outcomes with fewer safety events. Increased levels of the drug were associated with lower odds of a composite safety event at 28 days (odds ratio, 0.46; 95% CI, 0.33 to 0.63).
A higher-dose abatacept regimen would be necessary for most patients to achieve the exposure that resulted in optimal benefit.
"A higher-dose abatacept regimen would be necessary for most patients to achieve the exposure that resulted in optimal benefit derived from this ACTIV-1 IM cohort," the authors concluded.
US respiratory virus levels continue to decline as officials track shift in SARS-CoV-2 proportions
Respiratory virus activity continued to decline last week, and though COVID-19 indicators continue to decline, estimates of new variant proportions show some notable shifts in SARS-CoV-2, the Centers for Disease Control and Prevention (CDC) said in its weekly updates today.
.jpg)
In its weekly snapshot for flu, COVID-19, and respiratory syncytial virus (RSV), the CDC said no states are reporting high activity. Only one—North Dakota—is reporting moderate activity.
Flu markers continue to tail off
Flu activity continues to decline, with levels below baselines in all but 1 of 10 regions. Region 1, which includes six northeastern states, is at its regional baseline, the CDC said in its weekly FluView update .
Test positivity declined, and all three seasonal flu viruses continue to circulate, with influenza A making up 62.7% of positive samples at public health laboratories.
Hospitalizations declined, and overall deaths held steady. The CDC received reports of 6 more pediatric flu deaths, raising the season's total to 148. The deaths occurred from November 2023 to early this month. Three were due to influenza A, and three were linked to influenza B.
COVID trends decline, despite rise in FLiRT proportions
In its latest COVID data updates , the CDC reported further declines in its severity markers (hospitalizations and deaths), as well as its early indicators (test positivity and emergency department visits). Wastewater SARS-CoV-2 detections , another early indicator, remained at the minimal level and are at their lowest level since July 2023.
In its variant proportion update today, the CDC reported an ongoing decline in JN.1 variant detections and a steady rise in its spinoffs that have two added spike mutations, nicknamed the FLiRT (F for L at position 456 and R for T at position 346). The most notable rise was for KP.2, which edged above JN.1 and is now at 24.9%. Also, the proportion of another FLiRT subtype, called KP.1.1, rose from 3.1% to 7.5% over the past 2 weeks.
So far it's not clear if the FLiRT subtypes will trigger another rise in COVID activity.
USDA declares Salmonella an adulterant in raw breaded chicken products
The US Department of Agriculture (USDA) Food Safety and Inspection Service (FSIS) announced that it has finalized a policy to declare Salmonella an adulterant in raw breaded chicken products, which have been linked to 14 foodborne illness outbreaks that have sickened at least 200 people since 1998.
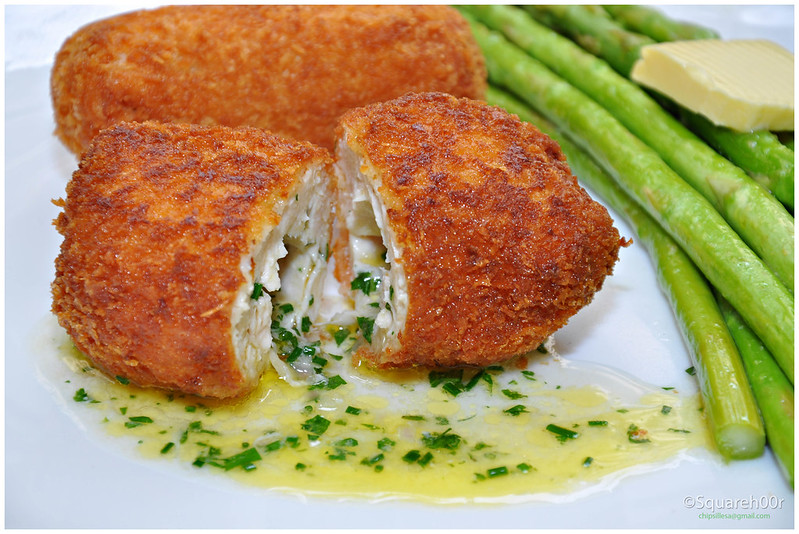
In its statement, the USDA said Salmonella would be considered an adulterant in the products if it exceeds a certain microbiologic threshold.
The step is part of a plan the agency proposed in 2022 to reduce Salmonella contamination in raw poultry products. FSIS added that it will address contamination in other raw poultry products later this year.
USDA Secretary Tom Vilsack said the final determination marks the first time Salmonella has been declared an adulterant in a class of raw poultry products. "This policy change is important because it will allow us to stop the sale of these products when we find levels of Salmonella contamination that could make people sick," he said.
It will allow us to stop the sale of these products when we find levels of Salmonella contamination that could make people sick.
FSIS said it would carry out testing procedures on the raw incoming chicken component prior to stuffing and breading to ensure that producers are controlling Salmonella in the products. It said the rule will go into effect 12 months after it is published in the Federal Register .
Raw breaded products present unique challenges
Though the raw breaded chicken products make up less than 0.15% of the domestic chicken market, they account for about 5% of all chicken-related foodborne illness outbreaks.
The products are problematic for consumers because they appear pre-browned and cooked. They are often cooked from a frozen state, which increases the chance that the raw chicken component may not reach the internal temperature needed to kill the Salmonella .
Texas officials confirm first cases of CWD in Edwards County
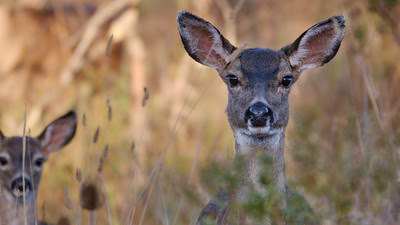
The Texas Parks and Wildlife Department (TPWD) and Texas Animal Health Commission (TAHC) yesterday announced the first detection of two cases of chronic wasting disease (CWD) in Edwards County, and further investigation revealed three more cases.
Two 2-year-old does in a deer-breeding facility tested positive at the Texas A&M Veterinary Medical Diagnostic Laboratory, and the National Veterinary Services Laboratory in Ames, Iowa, confirmed the finding. Subsequent testing of deer penned with the positive deer and other testing yielded three more cases.
CWD has been identified in Texas captive and free-ranging while-tailed deer, mule deer, red deer, and elk since it was first found in the state in 2012.
Infectious prion disease in cervids
Found in deer and other cervids such as moose and elk, the infectious illness is caused by misfolded proteins known as prions. The disease poses an ongoing threat to cervids, given that CWD can spread from animal to animal and through environmental contamination.
No human cases have been reported, but health officials urge against eating the meat of infected animals and to take precautions when field-dressing or butchering cervids.
Risk of uveitis recurrence higher in year after COVID vaccination
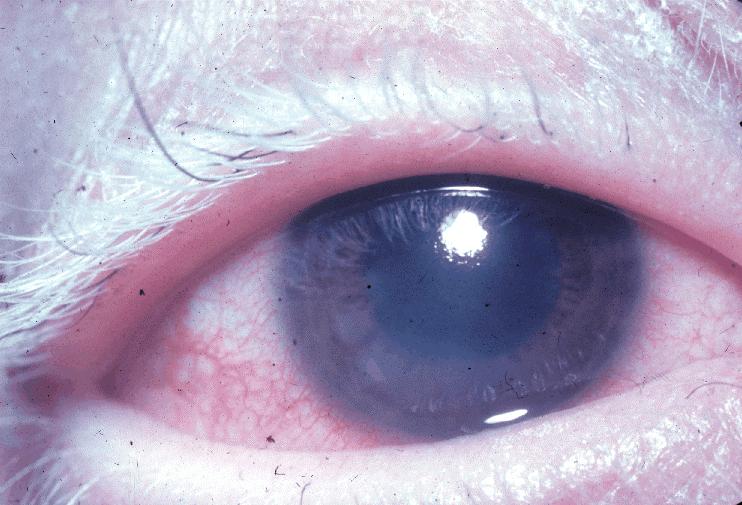
The incidence of uveitis in the year after COVID-19 was 17% among nearly 474,000 Korean adults with a history of the inflammatory eye condition, according to a report in JAMA Ophthalmology .
Researchers at the Hanyang University College of Medicine in Seoul mined the Korean National Health Insurance Service and Korea Disease Control and Prevention Agency databases for information on 473,934 patients diagnosed as having uveitis from January 2015 to February 2021.
The patients had previously had uveitis and had received at least one dose of an mRNA (Pfizer/BioNTech or Moderna) or adenovirus vector–based (AstraZeneca or Johnson & Johnson) COVID-19 vaccine. The average patient age was 58.9 years, 51.3% were women, and none tested positive for COVID-19 during the study period.
Uveitis is a potentially serious inflammation of the eye's middle layer of tissue that can cause symptoms such as pain, redness, and blurry vision.
Risk was elevated or all 4 vaccines
The incidence of uveitis was 8.6% at 3 months, 12.5% at 6 months, and 16.8% at 1 year. The odds of uveitis were increased among recipients of all four vaccines, including Pfizer (hazard ratio [HR], 1.68), Moderna (HR, 1.51), AstraZeneca (HR, 1.60), and Johnson & Johnson (HR, 2.07). The risk was highest in the first 30 days after vaccination and peaked between the first and second doses (HR, 1.64).
These results emphasize the importance of vigilance and monitoring for uveitis in the context of vaccinations, including COVID-19 vaccinations, particularly in individuals with a history of uveitis.
" Although uveitis following vaccination is rare, our findings support an increased risk after COVID-19 vaccination, particularly in the early postvaccination period," the study authors wrote. "These results emphasize the importance of vigilance and monitoring for uveitis in the context of vaccinations, including COVID-19 vaccinations, particularly in individuals with a history of uveitis. "
In a related commentary , Anika Kumar and Nisha Acharya, MD, said it's important to weigh the risk of uveitis with that of remaining unvaccinated against COVID-19. "Indeed, other investigations of postvaccine NIU [noninfectious uveitis] that similarly identified increased risks of NIU after vaccination noted that effect sizes were small and attributable risks were low; thus, the findings should not preclude individuals from receiving a vaccination," they wrote.
In case you missed it
This week's top reads, 1 in 5 us retail milk samples test positive for h5n1 avian flu fragments.
The highest concentrations were in regions where the virus has been found in dairy herds.

USDA scientists weigh avian flu vaccine for cows; virus may be spreading from cattle to poultry
Questions about transmission and clinical disease in cows need to be answered before vaccine development takes shape.
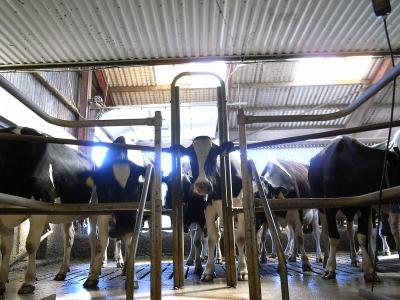
Global health groups propose new terminology for pathogens that spread through the air
The group steered away from defined cutoff points such as "droplets" and "aerosols" that scientists have used to distinguish larger particles from smaller particles.
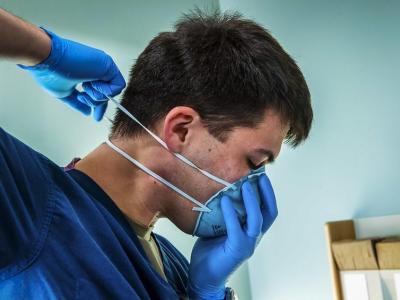
USDA shares recent H5N1 avian flu sequences amid more dairy herd outbreaks
In other developments, antiviral susceptibility testing shows that H5N1 is susceptible to neuraminidase inhibitor antiviral drugs.
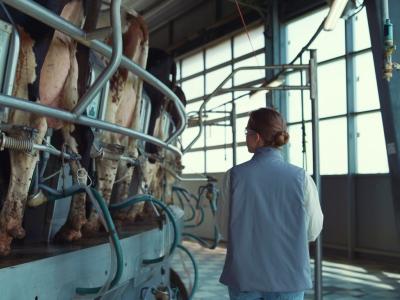
Tazewell County, Virginia reports first CWD case
The Virginia Department of Wildlife Resources confirmed the fatal prion disease in a buck harvested by a hunter in November 2023.
Scientists find clues in early analysis of newly shared US H5N1 avian flu sequences
Experts, with incomplete data, are quickly piecing together everything they can from the shared genetic sequences.
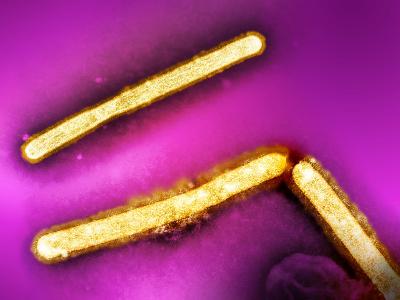
The odds of the condition were increased among recipients of all 4 vaccines studied: Pfizer, Moderna, AstraZeneca , and J&J.
COVID may have eroded doctors' belief that they are obligated to treat infectious patients
Reasons cited for accepting the option of refusing treatment included unreasonable risk to physicians and their families and labor rights and worker protection.
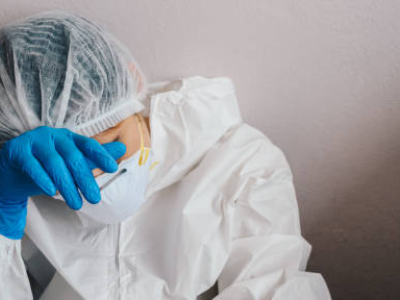
US orders testing for certain dairy cows after H5N1 avian flu remnants found in retail milk
The USDA says that lactating dairy cows must be tested before interstate transport.
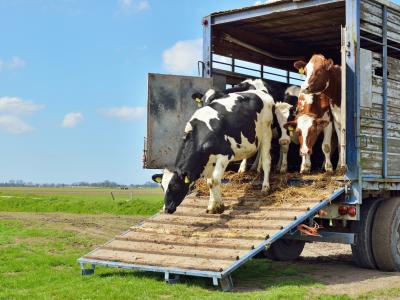
H5N1 avian flu infects Colorado dairy cows as global experts weigh in on virus changes
Global health groups say evolving developments, including a novel reassortant in Asia and increasing detections in mammals, require real-time monitoring.
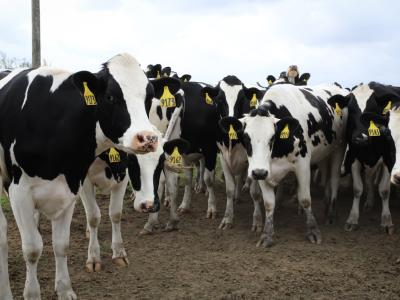
Our underwriters
Unrestricted financial support provided by.
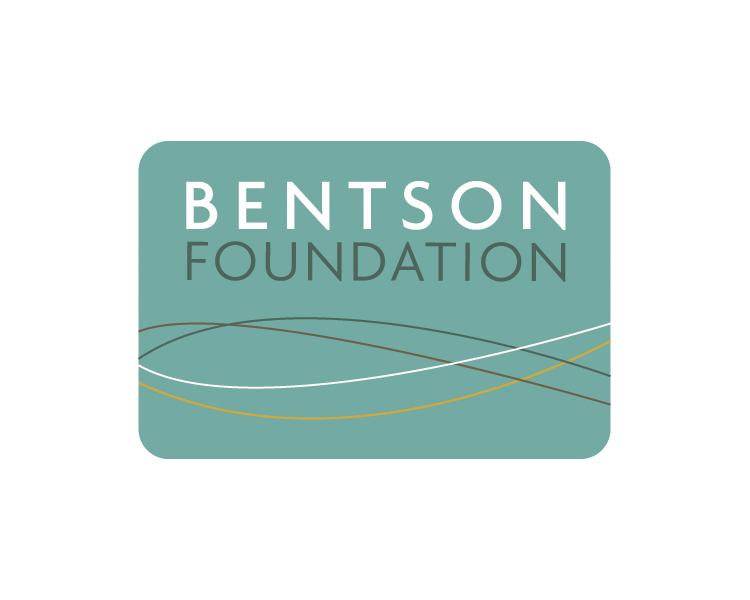
- Antimicrobial Resistance
- Chronic Wasting Disease
- All Topics A-Z
- Resilient Drug Supply
- Influenza Vaccines Roadmap
- CIDRAP Leadership Forum
- Roadmap Development
- Coronavirus Vaccines Roadmap
- Antimicrobial Stewardship
- Osterholm Update
- Newsletters
- About CIDRAP
- CIDRAP in the News
- Our Director
- Osterholm in the Press
- Shop Merchandise

An official website of the United States government
The .gov means it’s official. Federal government websites often end in .gov or .mil. Before sharing sensitive information, make sure you’re on a federal government site.
The site is secure. The https:// ensures that you are connecting to the official website and that any information you provide is encrypted and transmitted securely.
- Publications
- Account settings
Preview improvements coming to the PMC website in October 2024. Learn More or Try it out now .
- Advanced Search
- Journal List
- Rev Inst Med Trop Sao Paulo
- v.55(6); Nov-Dec 2013

Language: English | Portuguese
CASE STUDY OF A PATIENT WITH HIV-AIDS AND VISCERAL LEISHMANIASIS CO-INFECTION IN MULTIPLE EPISODES
Estudo de caso de paciente com múltiplos episódios da coinfecção hiv-aids e leishmaniose visceral, elis dionísio da silva.
1 Postgraduate Course of Biology applied to Health, Federal University of Pernambuco, PE, Brazil. E-mail: [email protected] , [email protected]
2 Graduate Course on Biological Sciences, Institute of Biological Sciences, University of Pernambuco, PE, Brazil
3 Parasitology Department, Aggeu Magalhães Research Center, Oswaldo Cruz Foundation, PE, Brazil. E-mails: rb.zurcoif.maqpc@said , rb.zurcoif.maqpc@eciremla , rb.zurcoif.maqpc@said , rb.zurcoif.maqpc@eciremla
Luiz Dias de Andrade
Paulo sérgio ramos de araújo.
4 Tropical Medicine Department, Clinical Hospital, Federal University of Pernambuco, PE, Brazil. E-mails: rb.zurcoif.maqpc@oigresp , rb.moc.lou@seahlagamev , rb.zurcoif.maqpc@oigresp , rb.moc.lou@seahlagamev
Vera Magalhães Silveira
Carlos eduardo padilha.
5 Service of Infectious and Parasitic Diseases, Clinical Hospital, Federal University of Pernambuco, Brazil. E-mail: moc.liamg@ahlidapgec , moc.liamg@ahlidapgec
Maria Almerice Lopes da Silva
Zulma maria de medeiros.
6 Pathology Department, Institute of Biological Sciences, University of Pernambuco, PE, Brazil. E-mail: rb.zurcoif.maqpc@soriedem , rb.zurcoif.maqpc@soriedem
Report of a 45-year-old male farmer, a resident in the forest zone of Pernambuco, who was diagnosed with human immunodeficiency virus (HIV) in 1999 and treated using antiretroviral (ARV) drugs. In 2005, the first episode of visceral leishmaniasis (VL), as assessed by parasitological diagnosis of bone marrow aspirate, was recorded. When admitted to the hospital, the patient presented fever, hepatosplenomegaly, weight loss, and diarrhea. Since then, six additional episodes of VL occurred, with a frequency rate of one per year (2005-2012, except in 2008). In 2011, the patient presented a disseminated skin lesion caused by the amastigotes of Leishmania , as identified by histopathological assessment of skin biopsy samples. In 2005, he was treated with N-methyl-glucamine-antimony and amphotericin B deoxycholate. However, since 2006 because of a reported toxicity, the drug of choice was liposomal amphotericin B. As recommended by the Ministry of Health, this report emphasizes the need for HIV patients living in VL endemic areas to include this parasitosis in their follow-up protocol, particularly after the first infection of VL.
Relato de caso de paciente masculino de 45 anos, agricultor, residente na zona da mata do Estado de Pernambuco, diagnosticado com HIV em 1999 e em uso de ARV. Em 2005 foi registrada a primeira ocorrência de LV através do diagnóstico parasitológico a partir do aspirado da medula óssea. À admissão no hospital apresentava-se com febre, hepatoesplenomegalia, perda de peso e diarréia. Desde então houve a ocorrência de mais sete episódios de LV, tendo ocorrido em media, um evento a cada ano (2005-2012 exceto em 2008). O paciente apresentou, em 2011, um quadro cutâneo disseminado, sendo realizada biopsia de pele que evidenciou formas amastigotas de Leishmania no exame histopatológico. Em 2005, o tratamento foi realizado com antimoniato de N-metil-glucamina e anfotericina B desoxicolato, mas desde 2006, devido à toxicidade, o medicamento de escolha foi a anfotericina B lipossomal. Como recomendado pelo Ministério da Saúde, esse relato reforça a necessidade de que os casos de HIV residentes em área endêmica de LV deverão ter inserido em seu protocolo de acompanhamento essa parasitose, principalmente após o primeiro episódio.
INTRODUCTION
Cases of visceral leishmaniasis (VL) co-infection with human immunodeficiency virus (HIV) and acquired immune deficiency syndrome AIDS (VL/HIV-AIDS) have been registered in 35 countries, mainly in southwestern Europe. VL/HIV-AIDS co-infections increase in areas where these two diseases coexist, as observed in Asian, African, and Latin American countries. In the latter group, Brazil has the highest number of cases 1 .
In 2011, in Brazil, VL appeared in 22 of the 27 Brazilian states, covering urban and suburban areas. Between 1998 and 2009, the annual average was 3,349 cases 7 . From 1980 to 2011 in Brazil, 608,230 cases of AIDS were reported. This epidemic tends to spread to poorly inhabited macro-regions as well as to medium and small cities 8 . When AIDS and VL databases were correlated, 176 cases of VL/HIV-AIDS co-infection were detected among the Federal States 7 .
Several episodes of VL are frequent in cases of VL/HIV-AIDS co-infection. According to BOURGEOIS et al. , 2010, these patients present a novel nosological entity called ‘active chronic visceral leishmaniasis’. This condition may be termed ‘chronic’ because of the presence of relapses over a period of several years and ‘active’ because of the continuous blood circulation of the parasite. On the other hand, it's impossible to know if repeated episodes are relapses or reinfections by using conventional parasitological and immunological methods 15 . Some studies show that individuals with HIV/AIDS and infected with VL often present atypical clinical manifestations and high incidence of relapse 5 22 24 25 . Molecular methods confirm that more than 90% of these cases are relapses, rather than reinfections 27 . The discrimination between relapses and reinfection can be made by molecular techniques based on restriction fragment length polymorphism (RFLP) analysis. The use of this technology may provide the physician with more information to determine Leishmania infections in patients who do not respond to treatment 20 .
Professionals, who treat patients with HIV/AIDS, report that this co-infection was not prioritized because of the variety of diseases related to immunosuppression, in addition to not being included among the AIDS-defining conditions 11 . To alert healthcare professionals about this association, we describe the case of a patient presenting multiple VL/HIV-AIDS co-infections during the seven years of evolution of this disease.
CASE REPORT
In 1999, a 31-year-old male farmer, who was a resident in the forest zone of Pernambuco, was admitted to the Clinics Hospital of the Federal University of Pernambuco. At the time of admission, he presented with asthenia and headache, and had diarrhea for at least 30 days. He was diagnosed with HIV, and began receiving antiretroviral therapy (ART) with stavudine, lamivudine, and efavirenz. Meanwhile, his 25-year-old partner and 9-month-old daughter were diagnosed with HIV infection.
Five years after initiating ART, the patient presented virological failure; after genotyping, his treatment was changed to tenofovir, lamivudine, and lopinavir/ritonavir. In 2005, he was diagnosed with VL as assessed by directly testing Leishmania in the bone marrow aspirate and initially treated with N-methyl-glucamine-antimony. However, because of pancreatitis, the patient began receiving amphotericin B, which was then replaced by a liposomal formulation because of the onset of renal failure.
In 2011, the patient presented disseminated cutaneous lesions caused by Leishmania , as assessed by histopathological analysis of skin biopsy samples. In July 2012, the patient was readmitted for presenting febrile disease with splenomegaly and pancytopenia, in addition to showing positive results for laboratory tests for leishmaniasis ( Table 1 ). After administration of liposomal amphotericin B deoxycholate, the patient's condition improved, and he was discharged upon recommendation of a secondary prophylaxis by administering liposomal amphotericin B twice a week. Between 2005 and 2012, seven VL infections occurred, as shown in the Table 1 .
HIV, human immunodeficiency virus; N.A., not available; N.D., not done; B.M. aspirate, bone marrow aspirate; d4T, stavudine; 3TC, lamivudine; EFV, efavirenz; TDF, tenofovir; LPVr, lopinavir/ritonavir; DAT, direct agglutination test; PCR, polymerase chain reaction.
This case describes some of the many clinical, diagnostic, and epidemiologic aspects of VL/HIV-AIDS co-infection. Immunosuppression caused by HIV might lead to the development of symptomatic VL 14 . In turn, VL might promote the clinical progression of HIV and of AIDS-defining conditions, thus, reducing the possibility of recovery after treatment and increasing the incidence of relapse 11 . This report showed that individuals with HIV/AIDS and living in endemic areas of VL should include VL assessment in their follow-up protocol. After the first co-infection, by means of clinical and laboratory support, a follow-up protocol of the patient should be created for early detection of relapse and re-infection.
One of the common features of co-infection is the increased tendency of relapse, observed in 37-80% of the patients 22 . Additionally, in some cases a chronic course with multiple occurrences might take place. This can be attributed not only to immunodeficiency but also to re-infection, host deficiencies correlating with ART, secondary prophylaxis, and CD4+ lymphocyte count 16 18 . CD4+ lymphocyte count is one of the most significant prognostic factors for survival 11 22 . VL usually appears as an opportunistic disease in HIV patients when CD4+ cell count is less than 200 cells/mm 3(1,6,12,13,17,25) . During the seven years of follow-up, the patient presented a CD4+ cell count ≤ 170 cells/mm 3 . This represents an important predictor of relapse. Relapses of VL are suggested to occur mainly in individuals with poor responses to antiretroviral treatment who have no improvement in CD4+ counts with a few exceptions 3 9 .
Based on clinical and biological [polymerase chain reaction (PCR)-based] follow-up, an ‘active chronic visceral leishmaniasis’ 5 has been proposed by BOURGEOIS et al. (2010). In our case, only the 6 th and 7 th episodes were able to have the peripheral blood (PB) analyzed by PCR, which showed positive results for Leishmania spp. As PCR-RFLP was only found in the 7 th sample episode, the etiological agent is Leishmania chagasi , according to the pattern of bands defined by SCHONIAN et al. (2003) 26 . Due to the absence of PB samples in previous episodes, the analysis by PCR-RFLP was not made. Therefore, it wasn't possible to distinguish between relapse and reinfection or characterize the case as ‘chronic visceral leishmaniasis’. Despite the medical importance of a clinical and laboratory monitoring of coinfected patients, this practice is still little used 12 19 20 .
ART plays an important role in reducing the effect of opportunistic diseases and in recent studies has shown a reduction in the incidence of VL. Studies in individuals with HIV/AIDS treated using ARV drugs showed a similar incidence of VL relapse when compared to studies of the pre-highly active antiretroviral therapy (HAART) era 11 14 . The increased survival resulting from ART might partially explain the high incidence of relapse observed in this population 18 . In the present study, during the eight years of follow-up, we observed seven VL infections, despite the patient receiving ART before the first infection.
VL manifestations associated with HIV infection might appear in a classical form, particularly in patients from VL-endemic areas, as well as with relatively aggressive symptoms that are sometimes non-specific and difficult to clinically diagnose 11 . In individuals with HIV/AIDS and presenting symptoms such as asthenia, anorexia, and weight loss, VL might be responsible for 7-23% of instances of fever of unknown origin 11 . This patient presented classic clinical manifestations during the study period, although in 2011, we observed the formation of skin lesions because of the parasite, as assessed by histopathological analysis.
Among the previously treated VL cases, several patients present a skin condition characterized by macular, popular, or nodular lesions, called Post-kala-azar dermal Leishmaniasis (PKDL) caused by the amastigotes of Leishmania donovani on the Indian subcontinent (India, Nepal, Bangladesh) and east Africa (Sudan, Ethiopia, Kenya) and caused by Leishmania chagasi in South America where it is rarely reported, as well as its presence in HIV positives 2 4 23 28 . It is worth noticing that exclusive involvement of the skin is an unusual condition, because the simultaneous appearance of skin lesions along with other VL manifestations was more frequently observed 21 . In this case, the skin lesion suggests a clinical PKDL, which developed five years after the first VL episodes, administration of multiple therapeutic regimens, and treatments of discontinuous secondary prophylaxis. Although it has been viewed amastigotes in biopsy specimens obtained from skin lesions, the hypothesis of PKDL can be suggested but not stated categorically because there was no characterization of Leishmania species involved in the cutaneous lesions, and may have been an infection of some sort cutaneous Leshmania endemic to the region as L. braziliensis .
Several studies on co-infected individuals show that they present a decrease in anti- Leishmania antibody levels in the peripheral blood 11 ; that is, in only 40-50% of VL/HIV-AIDS cases, specific antibodies are detected 1 . Conversely, assessment of Leishmania antigen in urine by latex agglutination test showed a sensitivity of 85-100% 1 . Polymerase chain reaction (PCR) in peripheral blood and bone marrow is a useful tool to diagnose, for follow-up, and detect relapses 22 . Although the literature shows that serological analyses are not the most convenient in patients presenting co-infection 1 6 , two serological tests (direct agglutination test and rK39-based rapid immunochromatographic test) performed enabled the diagnosis of such cases in 2011 and 2012. In the same years, latex agglutination test and PCR test showed positive results, thus, confirming the data in the literature. Similar to the finding in our study, CAVALCANTI et al. (2012), described a series of case studies of co-infection in the main hospitals of Recife, Brazil 10 .
There is currently sufficient evidence suggesting that secondary prophylaxis provides some protective effect but does not completely prevent the occurrence of relapse 11 . A meta-analysis study described that the average incidence of relapse in patients who did not receive secondary prophylaxis was 67%, whereas in those who received it was 31% 16 . Current recommendations from the Ministry of Health of Brazil 2 for the diagnosis, treatment, and follow-up of patients presenting co-infection state that the “efficacy of the secondary prophylaxis after the first successfully treated VL infection, was not completely established.” The suggested secondary prophylaxis ( Table 1 ) was poorly adopted, thus, compromising the clinical follow-up. Based on this case study and literature review, it is evident that co-infection presents typical clinical, diagnostic, and therapeutic features, and can be observed in the prognosis of the disease. Therefore, prospective studies are required to clarify gaps such as the efficacy of secondary prophylaxis and need for clinical and laboratory monitoring tools for the early assessment of relapse or re-infection.
Acknowledgments
This study was supported by the FACEPE/MS/CNPq Programme for Research and priority development to unified health system - SUS/ PCT Saúde II (project 03/2004) and CNPq/PIBIC/Fiocruz (process 139172/2012-2).
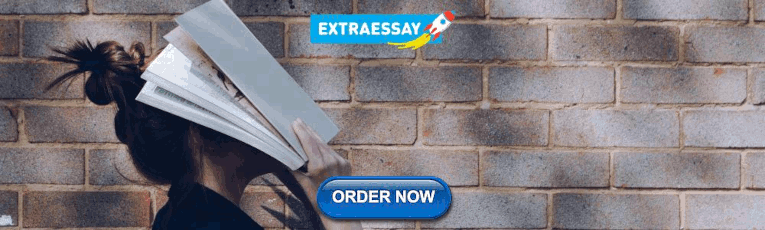
COMMENTS
A 68-year-old man was admitted to the hospital with fever, shortness of breath, and acute kidney injury. Testing of a nasopharyngeal swab for SARS-CoV-2 RNA was positive. Respiratory failure and hy...
Imaging studies show bilateral peripheral opacities. ... the epidemiologic characteristics of respiratory viruses (e.g., seasonal influenza) in order to appropriately consider viral causes of ...
Presentation of Case. Dr. Xavier Guell (Medicine): A 24-year-old man was transferred to this hospital because of fever, fatigue, cough, and dyspnea. The patient had been well until 5 days before ...
Technical guidance. Unity Studies: Early Investigation Protocols. Case management. National laboratories. Surveillance, rapid response teams, and case investigation. Infection prevention and control. Points of entry and mass gatherings. Naming the coronavirus disease (COVID-19) and the virus that causes it.
CASE REPORT. In January 2020, a 53-year-old gentleman with a background of asthma on long-term low dose inhaled corticosteroid inhaler had an acute exacerbation of his asthma in February 2020 triggered by a viral upper respiratory tract infection and acute sinusitis and was managed with bronchodilator nebulization and a 7-day course of oral prednisone 30 mg daily.
In severe cases, these increased levels can cause a cytokine storm and damages healthy tissue than the virus. 4,5. COVID-19 causes mostly fever, cough, sore throat, and shortness of breath, which in most cases are self-limiting. Some individuals harbor the virus and are asymptomatic. They play a crucial role in the spread of the virus in the ...
In a case series of 135 patients, Wan et al reported 88.9% of patients presented with a fever and 76.5% had a cough. Fatigue and ... Roques et al reported a study using chloroquine in Chikungunya virus reporting cytokines were reduced causing the adaptive immune response to be delayed, exacerbating fever, and unchanged suppression ...
Introduction. Viruses account for only a small fraction of the 1400 or more different species of pathogen that plague humans - the great majority are bacteria, fungi or helminths [].However, as both the continuing toll of childhood infections such as measles and recent experience of AIDS and influenza pandemics illustrate, viruses are rightly high on the list of global public health concerns [].
The study uses SARS-CoV-2 as a case study to present how the derived model could be used to characterize the virus propagation dynamics in humans. The main contributions of this study are as follows:
Genetic discordance of the two SARS-CoV-2 specimens was greater than could be accounted for by short-term in vivo evolution. These findings suggest that the patient was infected by SARS-CoV-2 on two separate occasions by a genetically distinct virus. Thus, previous exposure to SARS-CoV-2 might not guarantee total immunity in all cases. All individuals, whether previously diagnosed with COVID ...
"The rapid geographical expansion of [West Nile virus] between 2001-2002 is consistent with a large increase in virus genetic diversity (i.e., a 'polytomy,' as a result of many new ...
FRIDAY, April 19, 2024 (HealthDay News) -- An immune-compromised man with a year-and-a-half-long COVID infection served as a breeding ground for dozens of coronavirus mutations, a new study ...
Case Study: Prolonged Infectious SARS-CoV-2 Shedding from an Asymptomatic Immunocompromised Individual with Cancer Cell. 2020 Dec 23;183(7) :1901-1912 ... Our data indicate that certain immunocompromised individuals may shed infectious virus longer than previously recognized. Detection of subgenomic RNA is recommended in persistently SARS-CoV-2 ...
Real-Time Monitoring of Infectious Disease Outbreaks with a Combination of Google Trends Search Results and the Moving Epidemic Method: A Respiratory Syncytial Virus Case Study Trop Med Infect Dis . 2023 Jan 19;8(2):75. doi: 10.3390/tropicalmed8020075.
Check out 11 real cases of malware attacks. 1. CovidLock, ransomware, 2020. Fear in relation to the Coronavirus (COVID-19) has been widely exploited by cybercriminals. CovidLock ransomware is an example. This type of ransomware infects victims via malicious files promising to offer more information about the disease.
Summary. An outbreak of novel coronavirus (2019-nCoV) that began in Wuhan, China, has spread rapidly, with cases now confirmed in multiple countries. We report the first case of 2019-nCoV ...
Scientists have recently noted a connection between diabetes and the exposure to a virus. Coxsackieviruses are non-enveloped viruses with linear single-stranded RNA and are divided into group A and group B. Group A coxsackievirus tend to infect the skin and can cause hand foot and mouth disease. Group B tend to infect the heart and liver.
1 Background to the case study. Influenza is a myxovirus belonging to the family of viruses known as Orthomyxoviridae. The virus was originally confined to aquatic birds, but it made the transition to humans 6000-9000 years ago, coinciding with the rise of farming, animal husbandry and urbanisation.
Introduction. Human respiratory syncytial virus (RSV) is a single stranded RNA virus, a member of the family Pneumoviridae and genus Orthopneumovirus, and comprises two subgroups, A and B.Many studies have been published world wide regarding clinical features of RSV infection in pediatric, elderly, and immune-compromised adult populations in detail to demonstrate the clinical significance of ...
A 37-year-old man wounded by a wild monkey in Hong Kong is in intensive care suffering from infection with B virus.. Although this is the first reported case of a B virus infection in a human in ...
INTRODUCTION. The management of hepatitis B virus (HBV) infection is complex and depends upon multiple factors including clinical variables (eg, the presence or absence of liver inflammation and/or cirrhosis), the patient's immunologic response to infection (eg, hepatitis B e antigen status), virologic factors (eg, HBV DNA level and genotype ...
Find breaking science news and analysis from the world's leading research journal.
The aggregated case fatality rate associated with EBOV is 78%, which is higher than that for other Ebola virus species. 4,8,9 Here, we review the clinical course of two American health care ...
A n immune-compromised man with a year-and-a-half-long COVID-19 infection served as a breeding ground for dozens of coronavirus mutations, a new study discovered.. Worse, several of the mutations ...
April 19, 2024 - CDC continues to respond to the public health challenge posed by the outbreak of avian influenza A(H5N1) virus, or "H5N1 bird flu" in dairy cows and other animals in the U.S. CDC is collaborating with partners including the U.S. Department of Agriculture (USDA), the Food and Drug Administration (FDA), and state public health and animal health officials to address this ...
A study led by the University of Stirling jointly with the University of Wisconsin-Madison has shed new light on cross-species virus spillovers that can cause pandemics. Lab analysis of the bat ...
A new study shows good results in patients with severe COVID-19 for abatacept, an anti-inflammatory immunomodulating drug used to treat psoriatic arthritis, according to findings published yesterday in JAMA Network Open.. The study was based on results seen among 395 hospitalized patients in the ACTIV-1 IM randomized clinical trial, designed to assess the efficacy of abatacept, which inhibits ...
An outbreak of Nipah virus infection was confirmed in Kerala, India in May 2018. Five out of 23 cases including the first laboratory-confirmed case were treated at Baby Memorial Hospital (BMH), Kozhikode. The study describes the clinical characteristics and epidemiology of the Nipah virus outbreak at Kozhikode during May 2018.
Cases of visceral leishmaniasis (VL) co-infection with human immunodeficiency virus (HIV) and acquired immune deficiency syndrome AIDS (VL/HIV-AIDS) have been registered in 35 countries, mainly in southwestern Europe. ... thus, compromising the clinical follow-up. Based on this case study and literature review, it is evident that co-infection ...