A systematic review study on educational robotics and robots
- Related Documents
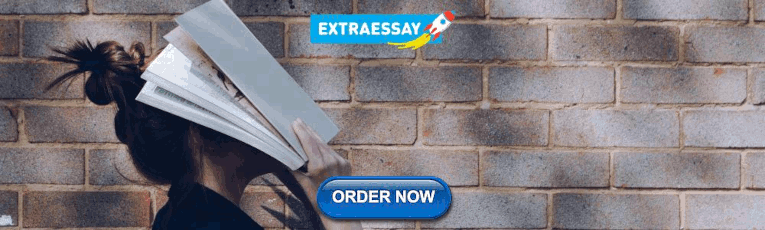
Influence of Occupant Behavior for Building Energy Conservation: A Systematic Review Study of Diverse Modeling and Simulation Approach
Energy consumption in buildings depends on several physical factors, including its physical characteristics, various building services systems/appliances used, and the outdoor environment. However, the occupants’ behavior that determines and regulates the building energy conservation also plays a critical role in the buildings’ energy performance. Compared to physical factors, there are relatively fewer studies on occupants’ behavior. This paper reports a systematic review analysis on occupant behavior and different modeling approaches using the Scopus and Science Direct databases. The comprehensive review study focuses on the current understanding of occupant behavior, existing behavior modeling approaches and their limitations, and key influential parameters on building energy conservation. Finally, the study identifies six significant research gaps for future development: occupant-centered space layout deployment; occupant behavior must be understood in the context of developing or low-income economies; there are higher numbers of quantitative occupant behavior studies than qualitative; the extensive use of survey or secondary data and the lack of real data used in model validation; behavior studies are required for diverse categories building; building information modeling (BIM) integration with existing occupant behavior modeling/simulation. These checklists of the gaps are beneficial for researchers to accomplish the future research in the built environment.
An investigation of the effects of environmental and ecologic factors on cutaneous leishmaniasis in the old world: a systematic review study
AbstractObjectivesLeishmaniasis is a neglected and widespread parasitic disease that can lead to serious health problems. The current review study aimed to synthesize the relationship between ecologic and environmental factors (e.g., weather conditions, climatology, temperature and topology) and the incidence of cutaneous leishmaniasis (CL) in the Old World.ContentA systematic review was conducted based on English, and Persian articles published from 2015 to 2020 in PubMed/Medline, Science Direct, Web of Science and Google Scholar. Keywords used to search articles were leishmaniasis, environmental factors, weather condition, soil, temperature, land cover, ecologic* and topogr*. All articles were selected and assessed for eligibility according to the titles or abstracts. The quality screening process of articles was carried out by two independent authors. The selected articles were checked according to the inclusion and exclusion criteria.Summary and outlookA total of 827 relevant records in 2015–2020 were searched and after evaluating the articles, 23 articles met the eligibility criteria; finally, 14 full-text articles were included in the systematic review. Two different categories of ecologic/environmental factors (weather conditions, temperature, rainfall/precipitation and humidity) and land characteristics (land cover, slope, elevation and altitude, earthquake and cattle sheds) were the most important factors associated with CL incidence.ConclusionsTemperature and rainfall play an important role in the seasonal cycle of CL as many CL cases occurred in arid and semiarid areas in the Old World. Moreover, given the findings of this study regarding the effect of weather conditions on CL, it can be concluded that designing an early warning system is necessary to predict the incidence of CL based on different weather conditions.
Systematic Review on Which Analytics and Learning Methodologies Are Applied in Primary and Secondary Education in the Learning of Robotics Sensors
Robotics technology has become increasingly common both for businesses and for private citizens. Primary and secondary schools, as a mirror of societal evolution, have increasingly integrated science, technology, engineering and math concepts into their curricula. Our research questions are: “In teaching robotics to primary and secondary school students, which pedagogical-methodological interventions result in better understanding and knowledge in the use of sensors in educational robotics?”, and “In teaching robotics to primary and secondary school students, which analytical methods related to Learning Analytics processes are proposed to analyze and reflect on students’ behavior in their learning of concepts and skills of sensors in educational robotics?”. To answer these questions, we have carried out a systematic review of the literature in the Web of Science and Scopus databases regarding robotics sensors in primary and secondary education, and Learning Analytics processes. We applied PRISMA methodology and reviewed a total of 24 articles. The results show a consensus about the use of the Learning by Doing and Project-Based Learning methodologies, including their different variations, as the most common methodology for achieving optimal engagement, motivation and performance in students’ learning. Finally, future lines of research are identified from this study.
What is the impact of multimorbidity on the risk of hospitalisation in older adults? A systematic review study protocol
IntroductionThe development of multiple coexisting chronic diseases (multimorbidity) is increasing globally, along with the percentage of older adults affected by it. Multimorbidity is associated with the concomitant use of multiple medications, a greater possibility of adverse effects, and increased risk of hospitalisation. Therefore, this systematic review study protocol aims to analyse the impact of multimorbidity on the occurrence of hospitalisation in older adults and assess whether this impact changes according to factors such as sex, age, institutionalisation and socioeconomic status. This study will also review the average length of hospital stay and the occurrence of hospital readmission.Methods and analysisA systematic review of the literature will be carried out using the PubMed, Embase and Scopus databases. The inclusion criteria will incorporate cross-sectional, cohort and case–control studies that analysed the association between multimorbidity (defined as the presence of ≥2 and/or ≥3 chronic conditions and complex multimorbidity) and hospitalisation (yes/no, days of hospitalisation and number of readmissions) in older adults (aged ≥60 years or >65 years). Effect measures will be quantified, including ORs, prevalence ratios, HRs and relative risk, along with their associated 95% CI. The overall aim of this study is to widen knowledge and to raise reflections about the association between multimorbidity and hospitalisation in older adults. Ultimately, its findings may contribute to improvements in public health policies resulting in cost reductions across healthcare systems.Ethics and disseminationEthical approval is not required. The results will be disseminated via submission for publication to a peer-reviewed journal when complete.PROSPERO registration numberCRD42021229328.
Approaches Consideration in the Management of Hepatitis (Warm E Kabid) by Means of Conventional Medicines and Herbal Drugs: A Systematic Review Study
Synthesis of fe-doped tio2 for photocatalytic processes under uv-visible light: effect of preparation methods on crystal size—a systematic review study, identifying occupational and non-occupational factors affecting the retention of health care employees in the covid-19 pandemic: a systematic review study, the challenge of designing ‘more’ experiential learning in higher education programs in the field of teacher education: a systematic review study, prevention, diagnosis, and treatment of covid-19 in infants and children: a systematic review study of performed protocols.
Context: Coronavirus disease 2019 (COVID-19) is one of the most dangerous viral diseases that started spreading in China in 2019 and has caused many deaths so far. Therefore, this study aimed to systematically review studies on the prevention, diagnosis, and treatment of coronavirus in infants and children. Evidence Acquisition: This is a systematic review study conducted in PubMed/Medline, Scopus, Embase, Cochrane Library, Science Direct, and Web of Science (ISI) databases on October 8 2020. Also, we searched Google Scholar to find all in-press articles. To extract data, a checklist was used, which included the author’s name, year of publication, purpose, age group of patients under study, and the protocol applied for coronavirus prevention, diagnosis, and treatment. The search was done with AND, OR, NOT strategies. The results of the study were reported in a descriptive manner using Endnote version 8 software. Results: According to the results of the basic search, 120 articles were extracted on the management of coronavirus. Of the articles extracted, 30 were articles related to children, of which only eight developed protocols for prevention, diagnosis, and treatment in infants and children. Of the studies extracted, six were from China, one from Iran, and one from Australia. It should be noted that the protocols for infants were extracted from two studies on infants and four studies on the pediatric group. Conclusions: It is proposed to consider and study this systematic review of coronavirus management in infants and children.
Phytotherapy in Renal Failure Due to Blood Pressure and Diabetes: A Systematic Review Study in Iram Ethnobotanical Documents
Export citation format, share document.
Journal of Pre-College Engineering Education Research (J-PEER)
Home > Libraries > LIBRARIESPUBLISHING > PUPOAJ > JPEER > Vol. 9 (2019) > Iss. 2
A Systematic Review of Studies on Educational Robotics
Saira Anwar , Purdue University Follow Nicholas Alexander Bascou , Rowan University Follow Muhsin Menekse , Purdue University Follow Asefeh Kardgar , Purdue University Follow
There has been a steady increase in the number of studies investigating educational robotics and its impact on academic and social skills of young learners. Educational robots are used both in and out of school environments to enhance K–12 students’ interest, engagement, and academic achievement in various fields of STEM education. Some prior studies show evidence for the general benefits of educational robotics as being effective in providing impactful learning experiences. However, there appears to be a need to determine the specific benefits which have been achieved through robotics implementation in K–12 formal and informal learning settings. In this study, we present a systematic review of the literature on K–12 educational robotics. Based on our review process with specific inclusion and exclusion criteria, and a repeatable method of systematic review, we found 147 studies published from the years 2000 to 2018. We classified these studies under five themes: (1) general effectiveness of educational robotics; (2) students’ learning and transfer skills; (3) creativity and motivation; (4) diversity and broadening participation; and (5) teachers’ professional development. The study outlines the research questions, presents the synthesis of literature, and discusses findings across themes. It also provides guidelines for educators, practitioners, and researchers in areas of educational robotics and STEM education, and presents dimensions of future research.
Recommended Citation
Anwar, S., Bascou, N. A., Menekse, M., & Kardgar, A. (2019). A Systematic Review of Studies on Educational Robotics. Journal of Pre-College Engineering Education Research (J-PEER), 9 (2), Article 2. https://doi.org/10.7771/2157-9288.1223
Since July 08, 2019
Included in
Educational Technology Commons , Engineering Education Commons , Robotics Commons , Science and Mathematics Education Commons , Science and Technology Studies Commons
To view the content in your browser, please download Adobe Reader or, alternately, you may Download the file to your hard drive.
NOTE: The latest versions of Adobe Reader do not support viewing PDF files within Firefox on Mac OS and if you are using a modern (Intel) Mac, there is no official plugin for viewing PDF files within the browser window.
ISSN: 2157-9288
- Journal Home
- Aims & Scope
- Editorial Board
- Author Rights
- Author Guidelines
- Reviewer Guidelines
- Submit Article
- Most Popular Papers
- Receive Email Notices or RSS
Advanced Search
Home | About | FAQ | My Account | Accessibility Statement
Privacy Copyright

A systematic review study on educational robotics and robots
11 citations
9 citations
3 citations
2 citations
1 citations
1,598 citations
846 citations
843 citations
471 citations
304 citations
Related Papers (5)
Ask Copilot
Related papers
Related topics
Advertisement
A Systematic Review on Exploring the Potential of Educational Robotics in Mathematics Education
- Published: 16 November 2018
- Volume 18 , pages 79–101, ( 2020 )
Cite this article
- Baichang Zhong 1 &
- Liying Xia 1
5284 Accesses
82 Citations
5 Altmetric
Explore all metrics
By providing students with a highly interactive and hands-on learning experience, robotics promises to inspire a new generation of mathematical learning. This paper aims to review the empirical evidence on the application of robotics in mathematics education and to define future research perspectives of robot-assisted mathematics education. After a systematic search in online database via keyword search and snowballing approach, we analyzed 20 empirical studies on how to teach and learn mathematical knowledge through robotics. The results indicate that (1) most studies were conducted with a small sample size, the largest research groups were elementary school students and secondary school students, most studies used LEGO robots, robots were primarily applied to teach and/or learn graphics, geometry, and algebra, and almost half of the studies taught mathematics by engaging students in game-like interactions with robots; (2) half of the studies adopted a non-experimental research design, and most studies evaluated student performance through observation, test/examination, questionnaires, or verbal interviews; and (3) instructional implications proposed in the 20 papers can be clustered into four themes: human-robot interaction, connections between mathematics and real life, pedagogical suggestions, and facility conditions. The 20 papers suggest that robotics generally plays an active role in mathematics education; however, there are indeed situations in which no significant improvement was found in students’ mathematical learning. In view of this, we prospect the future research perspectives of robot-assisted mathematics education and propose that more rigorous intervention studies could be conducted to further explore the integration of robotics and mathematics education.
This is a preview of subscription content, log in via an institution to check access.
Access this article
Price includes VAT (Russian Federation)
Instant access to the full article PDF.
Rent this article via DeepDyve
Institutional subscriptions
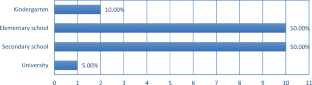
Similar content being viewed by others
How Have Robots Supported STEM Teaching?
Robotics in Education: A Smart and Innovative Approach to the Challenges of the 21st Century
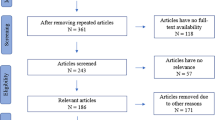
Systematic review: Potential effectiveness of educational robotics for 21st century skills development in young learners
Saira Bano, Kanwal Atif & Syed Atif Mehdi
Adams, K. D., & Cook, A. M. (2017). Performing mathematics activities with non-standard units of measurement using robots controlled via speech-generating devices: Three case studies. Disability and Rehabilitation: Assistive Technology, 12 (5), 491–503.
Google Scholar
Astleitner, H., & Wiesner, C. (2004). An integrated model of multimedia learning and motivation. Journal of Educational Multimedia and Hypermedia, 13 (1), 3–21.
Benitti, F. B. V. (2012). Exploring the educational potential of robotics in schools: A systematic review. Computers & Education, 58 (3), 978–988.
Article Google Scholar
Bers, M. U., Flannery, L., Kazakoff, E. R., & Sullivan, A. (2014). Computational thinking and tinkering: Exploration of an early childhood robotics curriculum. Computers & Education, 72 , 145–157.
Brown, L. N., & Howard, A. M. (2014). The positive effects of verbal encouragement in mathematics education using a social robot. In Proceedings of the 4th IEEE Integrated STEM Education Conference (pp. 1–5). Princeton, NJ: IEEE.
Ceci, S. J., & Williams, W. M. (2010). Sex differences in math-intensive fields. Current Directions in Psychological Science, 19 (5), 275–279.
Chen, G., Shen, J., Barth-Cohen, L., Jiang, S., Huang, X., & Eltoukhy, M. (2017). Assessing elementary students’ computational thinking in everyday reasoning and robotics programming. Computers & Education, 109 , 162–175.
Cheryan, S., Master, A., & Meltzoff, A. N. (2015). Cultural stereotypes as gatekeepers: Increasing girls’ interest in computer science and engineering by diversifying stereotypes. Frontiers in Psychology, 6 , 1–8.
Clements, D. H., & Meredith, J. S. (1993). Research on Logo: Effects and efficacy. Journal of Computing in Childhood Education, 4 (4), 263–290.
Fernandes, E., Fermé, E., & Oliveira, R. (2009). The robot race: Understanding proportionality as a function with robots in mathematics class. In V. Durand-Guerrier, S. Soury-Lavergne, & F. Arzarello (Eds.), Proceedings of the Sixth Congress of European Research in Mathematics Education (pp.1211–1220). Lyon, France: Institut National de Recherche Pedagogique.
Gomoll, A., Hmelo-Silver, C. E., Šabanović, S., & Francisco, M. (2016). Dragons, ladybugs, and softballs: Girls’ STEM engagement with human-centered robotics. Journal of Science Education and Technology, 25 (6), 899–914.
Hwang, W. Y., & Wu, S. Y. (2014). A case study of collaboration with multi-robots and its effect on children’s interaction. Interactive Learning Environments, 22 (4), 429–443.
Johnson, J. (2003). Children, robotics, and education. Artificial Life and Robotics, 7 (1), 16–21.
Julià, C., & Antolí, J. Ò. (2016). Spatial ability learning through educational robotics. International Journal of Technology and Design Education, 26 (2), 185–203.
Keren, G., Ben-David, A., & Fridin, M. (2012). Kindergarten Assistive Robotics (KAR) as a tool for spatial cognition development in pre-school education. In Proceedings of the 2012 IEEE/RSJ International Conference on Intelligent Robots and Systems (IROS) (pp. 1084–1089). Algarve, Portugal: IEEE.
Keren, G., & Fridin, M. (2014). Kindergarten Social Assistive Robot (KindSAR) for children’s geometric thinking and metacognitive development in preschool education: A pilot study. Computers in Human Behavior, 35 , 400–412.
Kitchenham, B. (2004). Procedures for performing systematic reviews. Keele, 33 , 1–26.
Kitchenham, B., Brereton, O. P., Budgen, D., Turner, M., Bailey, J., & Linkman, S. (2009). Systematic literature reviews in software engineering–a systematic literature review. Information and Software Technology, 51 (1), 7–15.
Kucuk, S., & Sisman, B. (2017). Behavioral patterns of elementary students and teachers in one-to-one robotics instruction. Computers & Education, 111 , 31–43.
La Paglia, F., La Cascia, C., Francomano, M. M., & La Barbera, D. (2017). Educational robotics to improve mathematical and metacognitive skills. Annual Review of CyberTherapy and Telemedicine, 15 (14), 70–75.
Leonard, J., Buss, A., Gamboa, R., Mitchell, M., Fashola, O. S., Hubert, T., & Almughyirah, S. (2016). Using robotics and game design to enhance children’s self-efficacy, STEM attitudes, and computational thinking skills. Journal of Science Education and Technology, 25 (6), 860–876.
Lindh, J., & Holgersson, T. (2007). Does LEGO training stimulate pupils’ ability to solve logical problems? Computers & Education, 49 (4), 1097–1111.
Mandin, S., De Simone, M., & Soury-Lavergne, S. (2017). Robot moves as tangible feedback in a mathematical game at primary school. Adv. Intell. Syst. Comput., 457 , 245–257.
Martin, F., Lurgio, M., & Coffey, D. (2006) Robotic jewelry: Inventing locally contextualized mathematics in a fourth grade classroom. In V. Dagiene & R. Mittermeir (Eds.), Proceedings of the Second International Conference on Informatics in Secondary Schools: Evolution and Perspectives (pp. 214–225). Vilnius, Lithuania: ISSEP.
Master, A., Cheryan, S., & Meltzoff, A. N. (2016). Computing whether she belongs: Stereotypes undermine girls’ interest and sense of belonging in computer science. Journal of Educational Psychology, 108 (3), 424–437.
Master, A., Cheryan, S., Moscatelli, A., & Meltzoff, A. N. (2017). Programming experience promotes higher STEM motivation among first-grade girls. Journal of Experimental Child Psychology, 160 , 92–106.
Menekse, M., Higashi, R., Schunn, C. D., & Baehr, E. (2017). The role of robotics teams’ collaboration quality on team performance in a robotics tournament. Journal of Engineering Education, 106 (4), 564–584.
Mitnik, R., Nussbaum, M., & Soto, A. (2008). An autonomous educational mobile robot mediator. Autonomous Robots, 25 (4), 367–382.
Mubin, O., Stevens, C. J., Shahid, S., Al Mahmud, A., & Dong, J. J. (2013). A review of the applicability of robots in education. Technology for Education and Learning, 1 , 1–7.
Nickels, M., & Cullen, C. J. (2017). Mathematical thinking and learning through robotics play for children with critical illness: The case of Amelia. Journal for Research in Mathematics Education, 48 (1), 22–77.
Nussbaum, M., & Soto, A. (2009). Collaborative robotic instruction: A graph teaching experience. Computers & Education, 53 (2), 330–342.
Padayachee, K., Gouws, P. M., & Lemmer, M. (2015). Evaluating the effectiveness of LEGO robots in engaged scholarship. In Proceedings of the Annual Global Online Conference on Information and Computer Technology (GOCICT) (pp. 16–20). Louisville, KY: IEEE.
Papert, S. (1980). Mindstorms: Children, computers, and powerful ideas . New York, NY: Basic Books, Inc.
Pinto, A. H., Tozadore, D. C., & Romero, R. A. (2015). A question game for children aiming the geometrical figures learning by using a humanoid robot. In Proceedings of the 12th Latin American Robotics Symposium and Third Brazilian Symposium on Robotics (pp. 228–233). Uberlandia, Brazil: IEEE.
Ponce, P., Molina, A., Hernández, L., Acha, E., Morales, B., & Huitron, C. (2017). Teaching math in elementary schools by LabVIEW and LEGO robots. Lecture Notes in Networks and Systems, 13 , 255–270.
Rhine, D., & Martin, F. (2008). Integrating mathematical analysis of sensors and motion in a mobile robotics course. Lecture Notes in Computer Science, 5090 , 41–52.
Rusk, N., Resnick, M., Berg, R., & Pezalla-Granlund, M. (2008). New pathways into robotics: Strategies for broadening participation. Journal of Science Education and Technology, 17 (1), 59–69.
Shankar, R., Ploger, D., Nemeth, A., & Hecht, S. A. (2013). Robotics: Enhancing pre-college mathematics learning with real-world examples. In Proceedings of the120th ASEE Annual Conference and Exposition (pp. 1–17). Atlanta, GA: ASEE.
Shih, B. Y., Chang, C. J., Chen, Y. H., Chen, C. Y., & Liang, Y. D. (2012). LEGO NXT information on test dimensionality using Kolb’s innovative learning cycle. Natural Hazards, 64 (2), 1527–1548.
Toh, L. P. E., Causo, A., Tzuo, P. W., Chen, I., & Yeo, S. H. (2016). A review on the use of robots in education and young children. Journal of Educational Technology & Society, 19 (2), 148–163.
Trochim, W. M. K., & Donnelly, J. P. (2006). Research methods knowledge base (3rd ed.). Mason: Thomson. Retrieved from http://www.socialresearchmethods.net/kb/ .
Walker, E., Girotto, V., Kim, Y., & Muldner, K. (2016). The effects of physical form and embodied action in a teachable robot for geometry learning. In J. M. Spector et al. (Eds.), Proceedings of the 16th International Conference on Advanced Learning Technologies (ICALT) (pp. 381–385). Austin, TX: CPS.
Wei, C. W., Hung, I. C., Lee, L., & Chen, N. S. (2011). A joyful classroom learning system with robot learning companion for children to learn mathematics multiplication. Turkish Online Journal of Educational Technology, 10 (2), 11–23.
Yelland, N. J. (1994). The strategies and interactions of young children in Logo tasks. Journal of Computer Assisted Learning, 10 (1), 33–49.
Download references
This work was financially supported by the “2011 Plan of Jiangsu: Collaborative Innovation Center for Strengthen Moral Education and Cultivate People, Nanjing Normal University”.
Author information
Authors and affiliations.
School of Education Science, Nanjing Normal University, No. 122, Ninghai Road, Nanjing, 210097, Jiangsu, China
Baichang Zhong & Liying Xia
You can also search for this author in PubMed Google Scholar
Corresponding author
Correspondence to Liying Xia .
Rights and permissions
Reprints and permissions
About this article
Zhong, B., Xia, L. A Systematic Review on Exploring the Potential of Educational Robotics in Mathematics Education. Int J of Sci and Math Educ 18 , 79–101 (2020). https://doi.org/10.1007/s10763-018-09939-y
Download citation
Received : 03 May 2018
Accepted : 05 November 2018
Published : 16 November 2018
Issue Date : January 2020
DOI : https://doi.org/10.1007/s10763-018-09939-y
Share this article
Anyone you share the following link with will be able to read this content:
Sorry, a shareable link is not currently available for this article.
Provided by the Springer Nature SharedIt content-sharing initiative
- Educational robotics
- Mathematics education
- Teaching/learning strategies
- Transdisciplinary issues
- Find a journal
- Publish with us
- Track your research
- No category
A systematic review study on educational robotics and robots
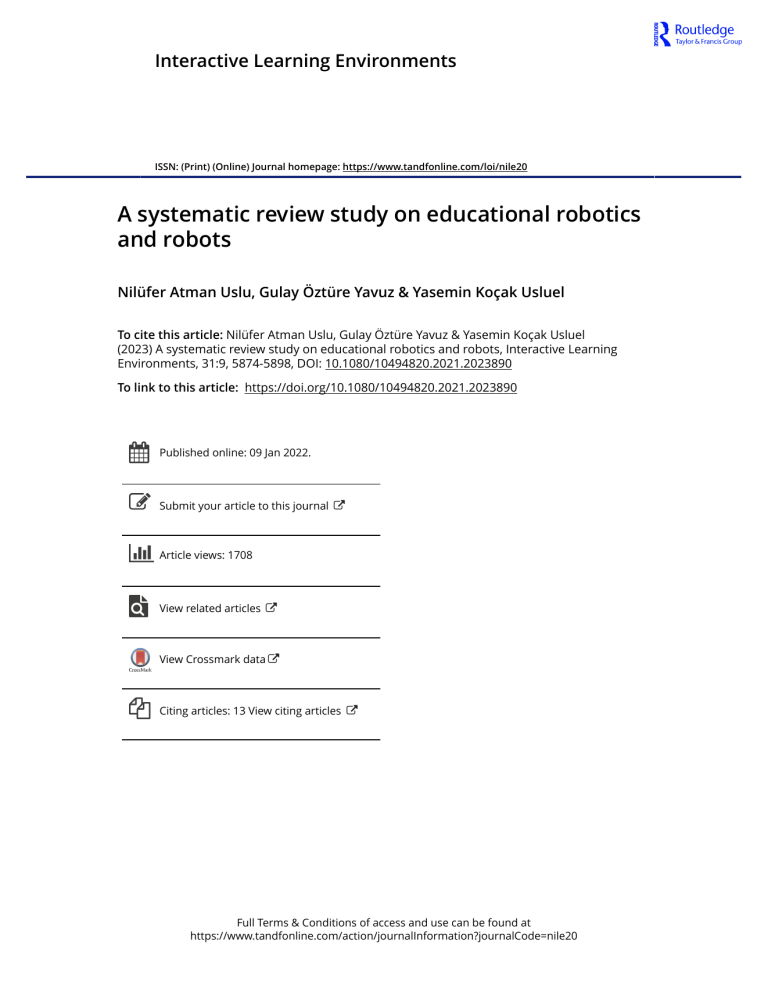
Related documents
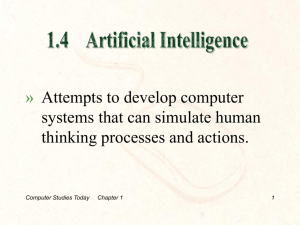
Add this document to collection(s)
You can add this document to your study collection(s)
Add this document to saved
You can add this document to your saved list
Suggest us how to improve StudyLib
(For complaints, use another form )
Input it if you want to receive answer
- Reference Manager
- Simple TEXT file
People also looked at
Systematic review article, educational robotics studies in italian scientific journals: a systematic review.
- 1 Department of Literature, Languages and Cultural Heritage, University of Cagliari, Cagliari, Italy
- 2 Department of Humanities, Philosophy and Education, University of Salerno, Salerno, Italy
In recent years, there has been increasing attention to applying educational robotics (ER) in learning settings and, consequently, it has concerned and involved the entire pedagogical field, giving rise to a large amount of experimentation and research. Educational robots are used within the school curriculum and in extra-curricular activities to improve student interest, engagement and academic achievement in various fields, such as STEM and digital literacy, and in many other ways, for example fostering specific cognitive and socio-relational skills. In Italy, as in many other countries, an increasing number of publications are featuring this subject. While there are also some reviews, none of them has been interested in reviewing studies published in Italian journals. The aim of this work is to provide a systematic review of the literature regarding studies investigating educational robotics and provide suggestions for further research and teaching practices. To do this we used the PRISMA statement process. In total, 28 studies published between 2011 and 2021 in 49 Italian journals were analyzed. The main findings from this review provide the current state of the art on research in ER. Furthermore, the paper discusses trends and the vision toward the future and opportunities for further research.
Introduction
Since from Papert's ( 1980 ) work, educational robotics has received global attention from the world of education, which has increasingly invested in this area of practice and research. Interest, which continues to grow to this day, has intensified over the last 10 years, as can be seen from the many literature reviews published on the international scene. These works highlight the breadth of the phenomenon, showing the different aspects and the varied research focuses. Benitti's ( 2012 ) review, one of the most cited, pointed out the potential of robotics in schools, highlighting the pedagogical features of projects but also the shortage of more quantitative work. The lack of meta-analysis can also be noted on this side. Mubin et al. ( 2013 ), in a review on the applicability of robotics in education, focused on the several practical aspects of using robots in education, addressing issues such as child-robot interaction and highlighting the added value of robots as stimulating and engaging teaching aids in the classroom.
Some authors have explored the use and teaching of robotics in the school context by highlighting the central role of the teacher who is required to act as a researcher to process new knowledge ( Papadakis et al., 2021 ; Tzagkaraki et al., 2021 ; Papadakis and Kalogiannakis, 2022 ). Although the potential of robotic technologies is widely recognized in the school context, some studies have investigated teachers' perceptions about the use of ER in preschools and elementary schools, and how these perceptions may affect the use of robots in educational practices ( Papadakis et al., 2021 ; Papadakis and Kalogiannakis, 2022 ).
The subjects examined by the researchers ranged from early childhood education ( Frison, 2019 ) to K-12 ( Karim et al., 2015 ; Xia and Zhong, 2018 ; Kyriazopoulos et al., 2021 ; Tzagkaraki et al., 2021 ), as well as young children ( Toh et al., 2016 ; Jung and Won, 2018 ). Additionally, they examined the learning environments in which such projects have been implemented: from the non-formal and extracurricular associative environment to the formal and curricular environment of school, including primary education ( Kyriazopoulos et al., 2021 ; Tzagkaraki et al., 2021 ). The richness, heterogeneity, and variety of work is explained by the fact that robots are intrinsically versatile tools. In fact, apparently, only hardware features define the affordance of the device. Many researches demonstrate that the multi-faceted nature of robotics fosters the design of countless new multidisciplinary activities in which technical-practical and social arguments coexist ( Anwar et al., 2019 ).
Research shows that robotics has also proved to be an important opportunity in inclusive education, distinguishing itself as a medium capable of providing effective support, in terms of wellbeing, attitude and knowledge, to socio-cultural disadvantage, special educational needs and qualify as a mediator and promoter of gender equality ( Daniela and Lytras, 2019 ; Sullivan and Bers, 2019 ; Bagattini and Miotti, 2022 ). In this vein, the synthesis by Pivetti et al. ( 2020 ) investigates studies on educational robotics to support children with neurodevelopmental disorders. Beyond the numerous studies that have classified multiple educational robots as particularly effective tools in the STEM disciplines ( Benitti, 2012 ; Toh et al., 2016 ), few of them have tried to evaluate the potential of robots with social-assistive attributes to foster understanding of scientific concepts ( Papadopoulos et al., 2020 ). In recent years, also in the field of social robotics, numerous studies have been conducted on the use of social robots to promote the development of specific skills in students, particularly for children with Autism Spectrum Disorder (ASD) ( Ferrari et al., 2009 ; Dunst et al., 2013 ; Conti et al., 2015 ), with the aim of promoting the development of social skills through verbal or non-verbal feedback. In educational settings, social robots are also used to fill the role of tutors or peers ( Belpaeme et al., 2018 ), supporting lessons and encouraging students to acquire new skills ( Leite et al., 2013 ), for example learning a second language. Although the use of educational robotics is widespread in each country and a variety of reviews are already available on specific aspects of the topic (see Table 1 ), we believe it is valuable to analyze specificities existing within a specific cultural context.
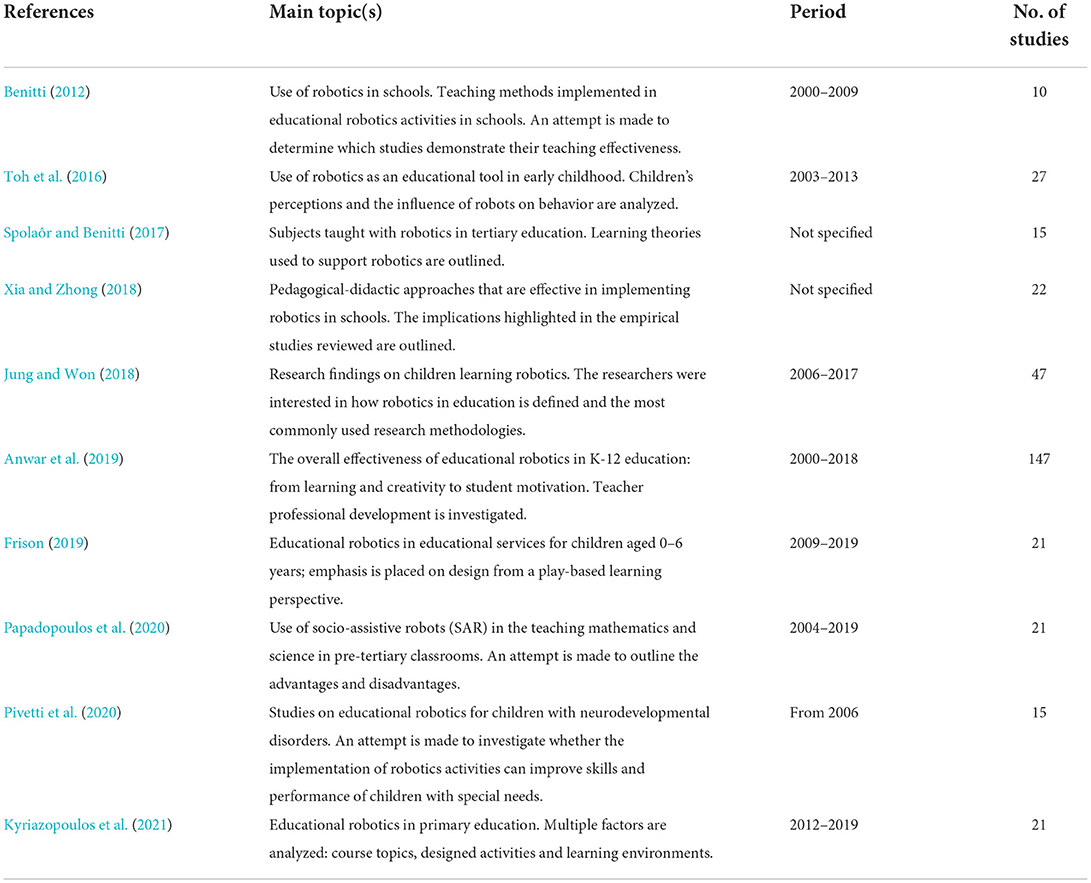
Table 1 . Systematic reviews of robotics in education.
This has driven us to undertake this systematic review of the research literature published in Italy over the past decade. This review has multiple purposes: to verify what types of experiences have been documented in Italian journals; to provide educational professionals and researchers with a reasoned summary of the work published to date, in order to understand the direction of research, what types of devices have been most widely tested in which educational settings, for what ages, and for the enhancement of which specific social, cognitive, motor skills; to provide researchers with directions that could facilitate the implementation of new robotics projects, with the intention of prompting new empirical research in order to enrich the scientific landscape 1 . Specifically, our research aims to examine the state of research in educational robotics based on the following research questions:
Question 1 : For what specific purposes are robots used in educational contexts, and how are these practices implemented (which teaching methods were used most)?
Question 2 : What are the general benefits of educational robotics according to the articles reviewed?
With the goal of capturing a clear picture of what has been published on the topic in the last 10 years in Italy, we have done a systematic literature review using Borrego et al. ( 2015 ) four-step process: search, selection, coding, and synthesis. The first two steps correspond to the stages of the PRISMA statement process—search (identification), selection (screening, eligibility, included)—while the other two concern the analysis work (coding and synthesis).
Search method
Since there is no specific database containing all the papers published in Italian journals, to retrieve the best Italian literature on robotics in education, it was necessary to find and search within each journal. Therefore, the first step was to carefully select the journals to be screened. For this purpose, we used the list of Class A journals 2 accredited by ANVUR, the Italian agency for the evaluation of academic research, selecting, in particular, those in the disciplinary area dealing with didactics, educational technology and educational research methodology (11/D2 sector). Forty-nine Italian journals were selected (see Appendix 1 ).
Articles were identified by working in two steps. First, using the Google search engine, a limited search was performed with the qualifier “site:journalname.it” (where “journalname.it” means the website of each journal identified). The search string used was the follow:
“ (Robot OR Robots OR Robotics OR Robotica) AND (coding OR pensiero computazionale OR computational thinking OR Social OR Sociale OR Student OR studente OR alunno OR School OR Scuola OR Education OR Educational OR Educazione OR Educativa OR inclusione OR inclusion OR Teaching OR Insegnamento OR Learning OR apprendimento OR didattica OR docente OR insegnante OR Formazione) .”
Subsequently, in order to check the completeness of the results, a search of each journal's website was executed using the same keywords. The lack of a specialized database for collecting articles published by Italian journals makes the task of compiling a research synthesis even more complicated. The search was performed from the beginning of February 2022 to the end of May 2022. A total of 122 papers were identified through Google search and websites.
Selection strategy
In the second phase, according to the PRISMA statement, the retrieved papers were analyzed based on the inclusion and exclusion criteria ( Table 2 ). Two of the authors of this study worked together on deciding to include or exclude a study. As already noted, a total of 122 papers were identified through Google search and websites ( Figure 1 ), of which 3 were reviews of other texts. Of these, 57 studies were discarded because, after reading their abstracts, they did not meet some of the identified inclusion criteria. The remaining 62 papers were independently evaluated by two authors who then compared them with the identified criteria and, after discussion, excluded an additional 34 papers, one of which was excluded because it was in Spanish.
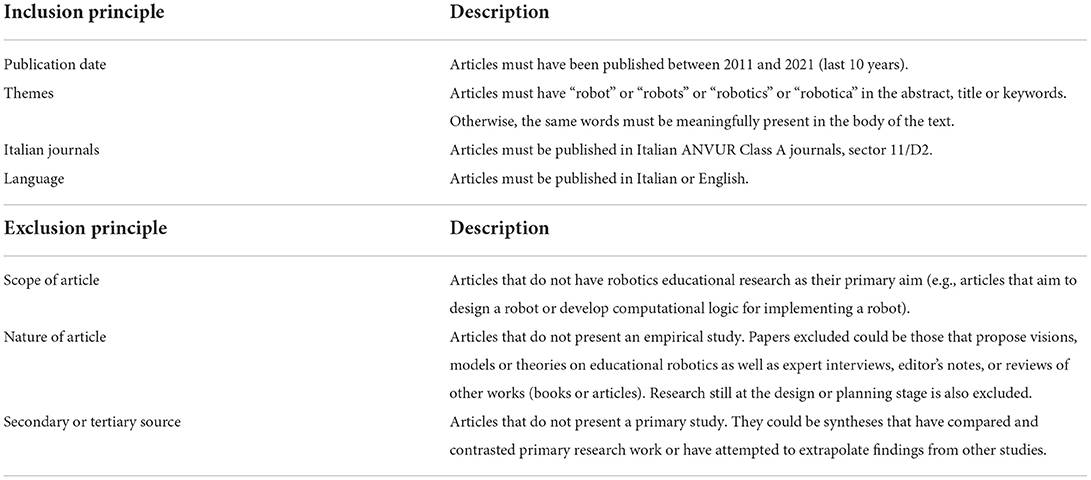
Table 2 . Criteria for the inclusion and exclusion of studies.
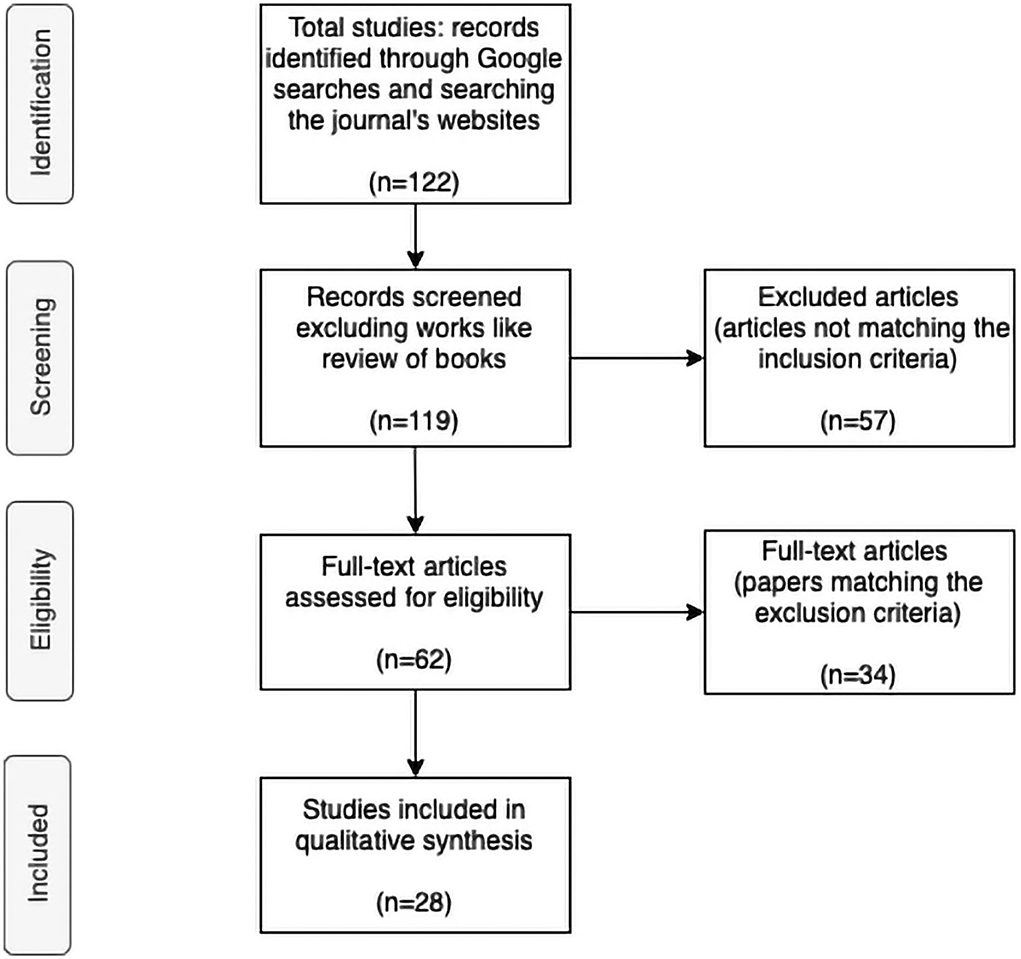
Figure 1 . Flow chart according to the PRISMA declaration ( Moher et al., 2019 ).
The diagram depicted in Figure 1 , based on the PRISMA declaration (Preferred Reporting Items for Systematic Reviews and Meta- Analyses), shows the entire process after which 28 papers were included in this review and subsequently thoroughly viewed and analyzed by the authors of this work. The papers included are listed in the “Results” section (see Table 3 ) and highlighted with an asterisk in the references.
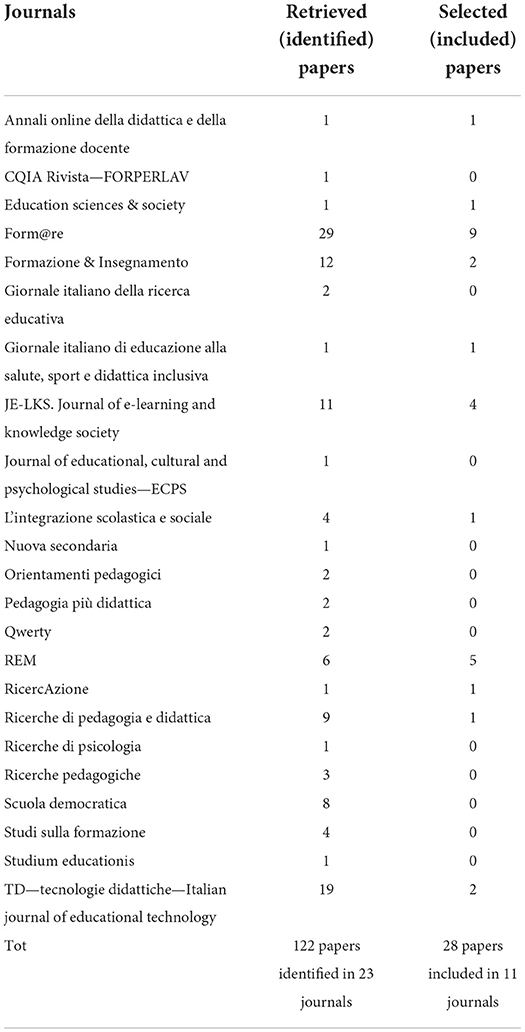
Table 3 . Papers identified and included in each Journal.
Coding and synthesis
In the third phase of the process, we collected relevant data and chose criteria to classify all studies. We arranged the 28 papers in a spreadsheet and listed them according to following information and features: (1) research method applied (qualitative, quantitative, mixed methods); (2) data collection used (observation, interview or focus group, test or assessment, questionnaire, student product evaluation); (3) Goals (education in robotics, education with robotics, teacher training, media education); (4) setting (formal, non-formal); (5) target (children, teenagers, adults); (6) school level (pre-school, primary school, middle school, high school, university); (7) subject (arts or humanities, life skills, inclusion, second language, STEM); (8) robot type (built by student, compact, social); (9) number of participants; (10) duration of empirical research. We then defined other categories, inferring them from reading the articles included and, in part, retrieving and adapting categories from other systematic reviews ( Bacca et al., 2014 ; Jung and Won, 2018 ). These categories may indicate some of the dimensions presented and analyzed in the research articles. Sometimes the information was not made explicit by the authors. Due to the lack of clarity, some authors' statements were interpreted. Some papers may provide data categorized into more than one subcategory (in which case, the categorizations in the tables of this paper have an asterisk symbol to the right of the category name). In the last stage, synthesis, we summarized the main findings of the selected articles, answering the research questions.
The papers analyzed were published from 2011 to 2021. Studies published in 2022 were not taken into account due to the publication time of this work. To date, different studies on the topic are “pending publication,” and they are not yet fully available; therefore, in this work, any papers from 2022 have not been evaluated. Figure 2 shows the trend in publications within the time range analyzed. As the graph shows, there is a publication spike in 2019. This variation is due to the publication of a special issue of the journal Form@re on the topic of robotics.
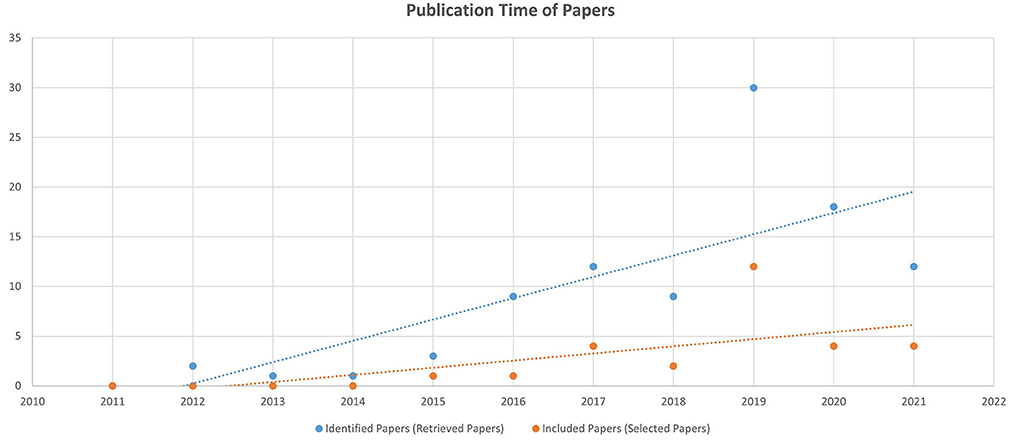
Figure 2 . Retrieved and selected papers.
Our search, which considered 49 Italian journals, led to identify 122 papers in 23 journals and, after the analysis and evaluation phases, to select 28 papers from 11 different journals (see Table 3 ). Remarkably, in 26 Italian journals, educational robotics issues it seems that are never considered.
In the Table 4 we summarize main design features of 28 papers included.
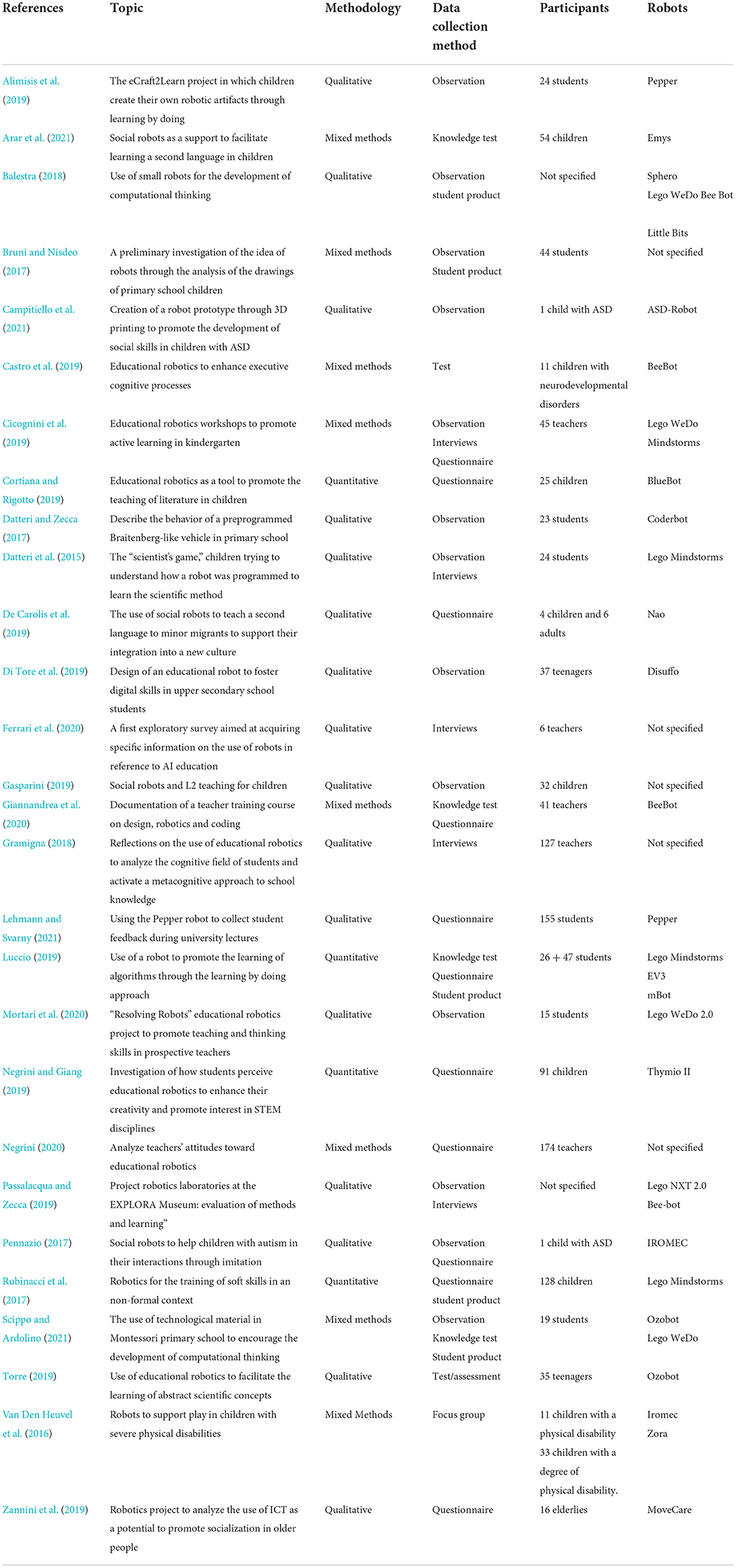
Table 4 . Articles selected and study type.
Table 5 reports the main features that characterize the selected research papers.
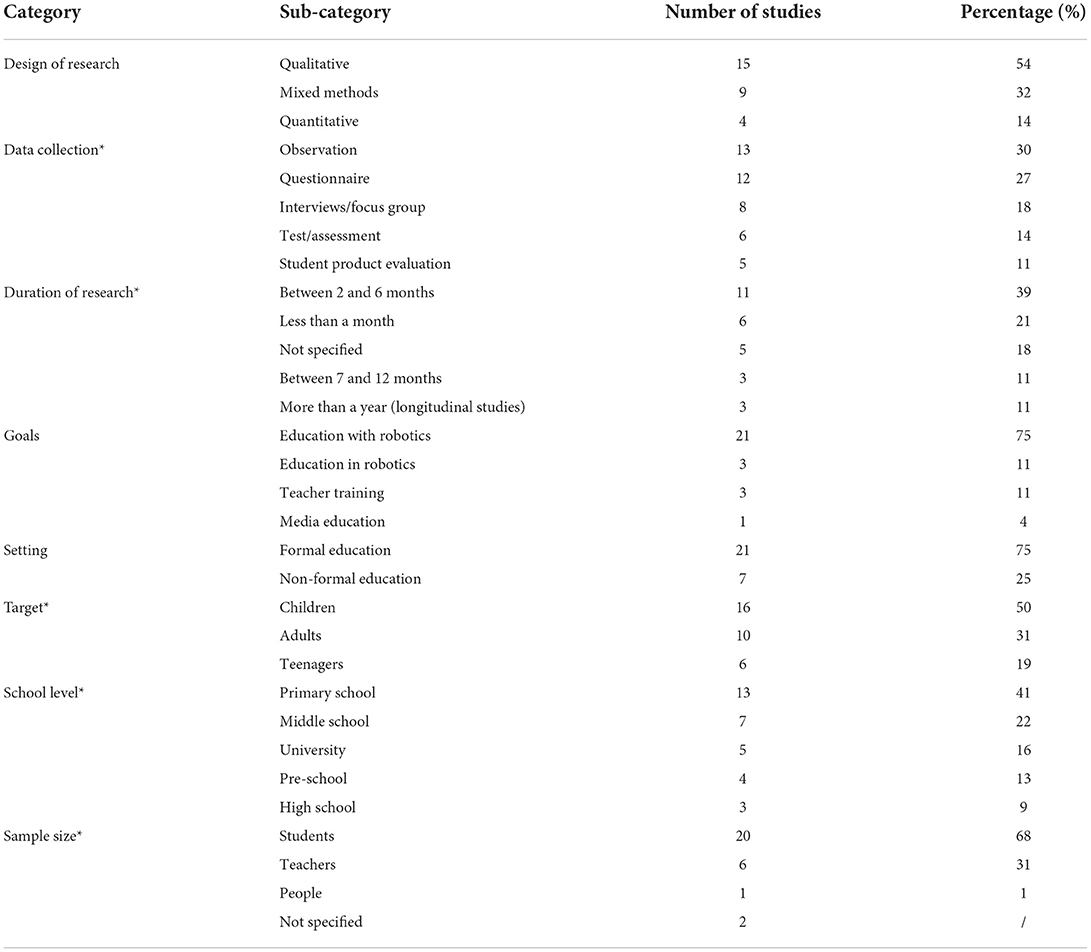
Table 5 . Summary of research data.
Based on the data summarized by the table above, we can state that:
• the preferred type of research design was qualitative (54%);
• the preferred type of data collection was observation (30%);
• the average duration of the research analyzed was around 6 months;
• 75% of the research has focused on the goal of education with robotics;
• 75% of the research has preferred a formal setting;
• most of research was conducted with students attending primary school;
• the average sample was composed of 49 subjects.
The samples retrieved from the analyzed research broken down in Table 6 .

Table 6 . Descriptive sample statistics.
According to the data shown in the table above, the sample size varies greatly, as summarized by the variation coefficient (1,2). This variation is partially due to the type of research, with the case studies on inclusion and disabilities having small samples. It is also interesting that two studies (7% of total analyzed studies) do not give any information about their samples. With respect to subjects, most of the research was aimed at fostering the development of STEM competencies, as Table 7 illustrates.
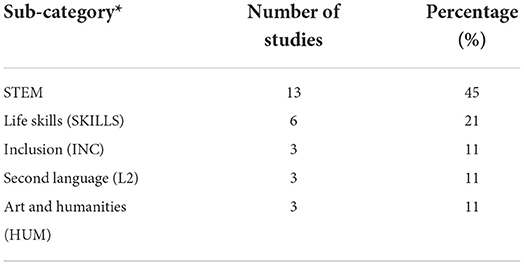
Table 7 . Area of knowledge/subjects.
To answer the second research question, aimed at identifying the general benefits of educational robotics, we attempted to isolate and summarize the main educational objectives of the works through a categorization of the dimensions analyzed (see Table 8 ). Such dimensions (problem-solving, motivation, inclusion, etc.) point out the authors' interest in some aspects related to specific expected benefits through the practice of educational robotics. However, as we shall see in the discussion, in several cases the data stop at mere statements of principle without adequately supporting them with empirical data or arguments.
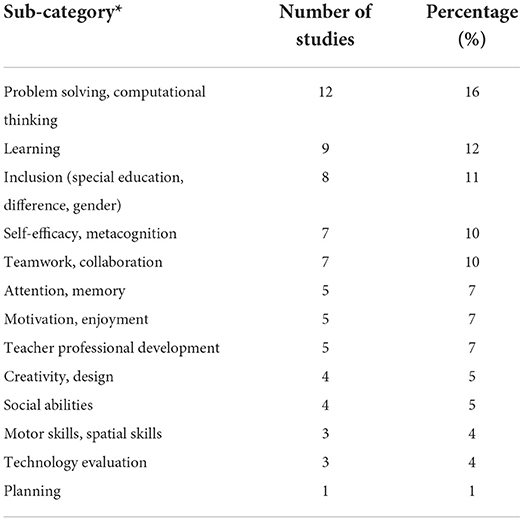
Table 8 . Dimensions analyzed.
As Table 8 illustrates, most of the studies were conducted with the aim of fostering the development of computational thinking (16%), while only 4% of studies were conducted with the aim of evaluating the technology from a usability, effectiveness, acceptability, or sustainability point of view. Two researchers independently coded the papers in order to categorize them. The rules were developed through discussions between the authors of this paper. Interrater reliability (Cohen's Kappa) was strong (k = 0.89), and disagreements between raters were all resolved through discussion.
Aggregating the data from Table 8 we can see that 45% of the analyzed papers focused on fostering the development of metacognition processes and 26% were related to inclusion and social topics. Only 7% of the studies were conducted to foster teachers' professional development.
As Table 9 shows, most of the studies (28%) were conducted using a project-based approach as teaching method, while only 7% of the studies were conducted using a free-play-approach. Most of them were organized as group work.
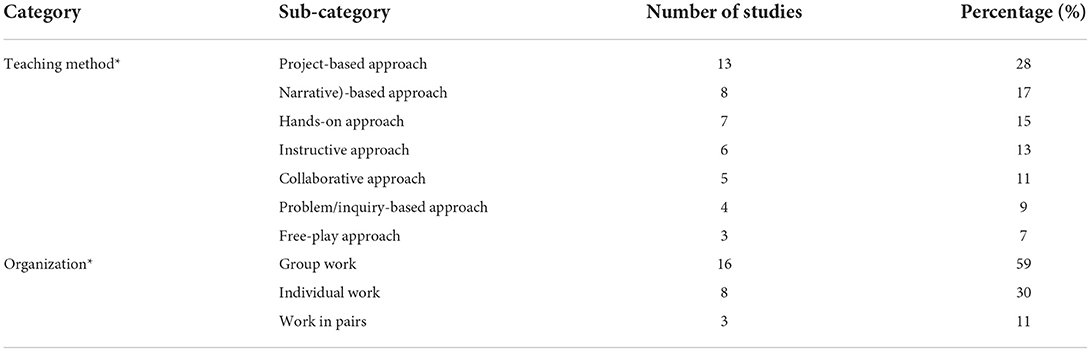
Table 9 . Classroom work.
Table 10 shows that most of the studies (82%) used robotic hardware. Of the total studies included, 17 (61%) used an educational robot, within that number, 3 studies used built and compact robots, and for this reason they were calculated twice within the subcategory. Only 6 researches (21%) used a social robot, and 5 researches (18%) did not use any robotic devices.

Table 10 . Robot types.
Based on the research questions that guided our investigation, we must point out the limitations of this work and suggest new frontiers of research on educational robotics. Of the 28 papers accepted published between 2011 and 2021, 26 were published in the last 5 years and 12 in 2019 alone. These data allow us to surmise that, also in Italy (see Figure 2 ), there has been growing interest in robotics, confirmed by the scientific literature. This is in line with the references of several researchers ( Sullivan and Bers, 2019 ; Ching et al., 2019 ; Kyriazopoulos et al., 2021 ) regarding the lack of research on robotics (before 2017), a trend that does not reflect the growth observed in recent years.
Main findings
Our work, the main aim of which was to retrieve and analyze articles published in Italian Class A journals on the subject of educational robotics over the last 10 years (2011–2021), sought to answer two main research questions that guided the investigation from the outset. Regarding the first question, namely “For what specific purposes are robots used in educational contexts, and how are these practices implemented (which teaching methods were used most)?”, we can state that we are in line with what has been reported in the international literature (e.g., Benitti, 2012 ; Anwar et al., 2019 ; Kyriazopoulos et al., 2021 ), i.e., that the main reason for its adoption is related to the promotion of STEAM skills. A series of Italian works have stated the potential of robotics in educational competitions to promote the development of problem-solving skills and computational thinking ( Balestra, 2018 ; Luccio, 2019 ; Mortari et al., 2020 ; Scippo and Ardolino, 2021 ), soft skills ( Rubinacci et al., 2017 ) and disciplinary topics ( Cortiana and Rigotto, 2019 ; Torre, 2019 ). Torre ( 2019 ) introduces math reasoning in high school in an interdisciplinary way through examination of dilemmas in a literary tale. The proposed story involves different time trajectories for the various protagonists to be achieved through problem solving and robot programming. Educational robots are also used to promote the development of scientific thinking (investigating the ability to intuit the behavior of a robot) and the use of scientific method ( Tzagkaraki et al., 2021 ) using an inquiry-based approach ( Datteri et al., 2015 ; Datteri and Zecca, 2017 ). A type of robot called “MoveCare,” on the other hand, has been used to improve the autonomy of elderly people ( Zannini et al., 2019 ), confirming that the potential purposes of robotics are multiple and ever-expanding.
Social robots are also present in the literature investigated in the field of special education, either to help children with ASD in social interactions by observing behavior and using human-robot imitative modeling to support the development of basic social skills ( Pennazio, 2017 ; Campitiello et al., 2021 ), or as in the case of Iromec and Zora ( Van Den Heuvel et al., 2016 ), to support play in children with severe motor disabilities. Robots with social features have taken on the role of tutors in teaching a second language ( De Carolis et al., 2019 ; Gasparini, 2019 ; Arar et al., 2021 ). De Carolis et al. ( 2019 ) explore how social robots (specifically NAO) can be used in teaching a second language to unaccompanied migrant children. The overall aim of this research is to support the cultural processes of inclusion. The work has given ample space to the cultural aspect of the gestures required to enable social inclusion. Gasparini ( 2019 ) presents a preliminary study on the use of the social robot NAO for teaching L2 vocabulary through the selection of gestures that can accompany comprehension. However, the work does not yet provide direct evidence. Lehmann and Svarny ( 2021 ) were interested in probing students' thoughts and opinions on the role of Pepper, a humanoid social robot, in providing learning support during university lectures. The authors also wondered which type of feedback from the robot was most productive.
Some work focused on technology evaluation and measured whether the robotic technologies designed and implemented were sustainable and/or accepted by teachers and students. Two examples are works on the alpha and beta testing phases of designed and 3D-printed artifacts: a built robot ( Di Tore et al., 2019 ) to develop digital skills in high school and a social robot ( Campitiello et al., 2021 ) to foster the development of social skills in children with ASD. A considerable number of studies analyzed the aspect of teacher and student perceptions of robotics resulting from the use and implementation of this practice at school. In general, this type of research has been conducted through the use of questionnaires or interviews. There are at least five papers ( Gramigna, 2018 ; Cicognini et al., 2019 ; Ferrari et al., 2020 ; Giannandrea et al., 2020 ; Negrini, 2020 ) that, overall, show that teachers have a strong interest in robotics. Regarding students' perceptions of educational robotics, Negrini and Giang ( 2019 ) investigated its pedagogical value in formal education. Following a semester of workshops with Thymio II, four dimensions were investigated through a questionnaire: creativity, collaboration, computer skills (computational thinking), and promotion of interest in STEM. The work of Rubinacci et al. ( 2017 ) also aims to investigate the expectations and opinions of students and teachers with respect to the development of soft skills, teamwork, understanding and problem solving.
Overall, based on our findings, we can state that the research published in Italian journals is moving in different directions, but the environment in which the experience takes place, in most of the works, appears to be formal. This finding helps us answer the second part of the question, which aims to examine which teaching methods were used when the research involved students. The results show a variety of methodological approaches (see Table 9 ) in the implementation of these practices, aimed at making the role of the student active and the teacher a facilitator ( Tzagkaraki et al., 2021 ). Often, the teaching method was not explained in the articles, so it was difficult for the authors to determine which method accompanied these experiences. When the child-robot interaction was free and there was no instructional approach, the authors interpreted it as free play. In many works, however, it seems possible to interpret an eclectic use of multiple methodologies. Many educational robotics activities for students are supported by collaborative architectures. In 10% of them, working in pairs was preferred ( Pennazio, 2017 ; Rubinacci et al., 2017 ; Scippo and Ardolino, 2021 ), while in 13% the working in a group was open ( Balestra, 2018 ; Giannandrea et al., 2020 ; Arar et al., 2021 ; Lehmann and Svarny, 2021 ), even to different age groups, which may mean the coexistence of other methodologies such as peer tutoring. Indeed, it would seem that the collaborative approach can be put into practice in different ways and is sometimes supported by other methodologies and, in particular, exploratory architectures.
The second research question in this review seeks to define the general benefits of robotics in the educational environment and the evidence on which they are based. Arar et al. ( 2021 ), in the social robotic area, have provided reliable results indicating that the use of a social robot in children's L2 learning increases the effectiveness of the educational process and significantly improves learning gains. De Carolis et al. ( 2019 ), in a preliminary study, show the effectiveness of the proposed approach in learning gestures using a social robot as well. The interaction was found to be engaging and quite spontaneous. Pennazio ( 2017 ) pilot study notes the robot's ability to promote the acquisition of social skills through imitation and interaction. The author reports an increase in different measures (e.g., eye contact and attention) and a smooth transition in communication aspects. The child moves from seeking contact (e.g., caresses, hugs) from the robot to contact with the teacher and then with peers. Van Den Heuvel et al. ( 2016 ) evaluated the usability and playfulness of the Zora and Iromec robots with children with severe disabilities and found a significant and promising contribution in supporting free play and achievement of predetermined goals in rehabilitation and special education. Regarding teachers' perceptions, Negrini's ( 2020 ) results show an interest and propensity to use robots for soft skills development. The study highlights factors that teachers believe limit the implementation of robotics in the classroom (e.g., cost and instructional design time). However, they prefer to use robots as educational technology tools to be programmed rather than as social robots that function as tutors or assistants. Rubinacci et al. ( 2017 ) also noted that teachers see robotics as a useful tool for promoting soft skills, although, it is unclear whether it is the laboratory as an experience rather than the use of robots that develops these skills. The research also reports that students rated the lab positively in terms of promoting technical skills. In terms of enhancing soft skills, it emerges that students have high expectations for teamwork, understanding and problem solving. Importantly, for students in the post-test, the perceived effectiveness of robotics in supporting teamwork skills decreases. Negrini and Giang ( 2019 ) showed that students were generally interested in working with the robot and believed that educational robotics enhanced twenty-first century skills. However, the greatest perceived improvements were found in the two dimensions of soft skills (i.e., collaboration and creativity) and not in the two dimensions of technical skills (i.e., computational thinking and computer science). The study on children's imagery by Bruni and Nisdeo ( 2017 ) shows as overall results a strong prevalence of an anthropomorphic view of the robot. Scippo and Ardolino ( 2021 ), through a longitudinal study and a mixed-method approach, observed an increase in creativity, sense of community, good self-efficacy, and low error pervasiveness after a course with the robot Ozobot. Cortiana and Rigotto ( 2019 ), on the other hand, noted the usefulness of ER in encouraging readers' identification with characters proposed by literary texts. In an experiment conducted in a primary school, through pre-post administration of an adaptation of a validated questionnaire, they showed that robotics supports the development of affective-emotional dimensions by making the student more engaged and motivated. Significant change was shown in the area related to pleasure associated with reading. Two works ( Datteri et al., 2015 ; Datteri and Zecca, 2017 ) were interested in understanding the development of students' scientific thinking through the use of robots. The authors, analyzing student transcripts, found a category-structured taxonomy for assessing children's modes of explanation, useful for teachers to make an analysis of the cognitive skills employed during robotics activities. Castro et al. ( 2019 ) have also experimented with educational robotics in school settings. Various tests were administered before and after the workshops, which showed improvement in executive functions (e.g., inhibition). Luccio ( 2019 ) focused on performance, showing that the hands-on robot programming project significantly improved exam performance. From the test results on student performance, it was argued that, in general, educational robotics supported by an instructional methodology, such as the project-based approach, can support the learning process by making it more interesting, increasing collaboration skills and also the very knowledge of theoretical concepts.
Limitations
This study has several limitations. Primarily, although a thorough search strategy was used, some empirical studies on the use of ER may not have been found for several reasons: the lack of a single, reliable search engine capable of indexing all Italian journals; the fact that not all publishers publish articles online in digital format (or that they must be paid for); the fact that some journals have changed publishers over time, leaving the repositories partially incomplete. The search for studies also did not cover either chapter in volumes of texts on robotics or conference proceedings. Secondly, several of the included studies showed, on one hand, a lack of information and data clarifying the implemented research design and, on the other, methodological weaknesses. In fact, very few studies presented a strict experimental-type structure. The methodological nature, therefore, included the possibility of being able to trace the evidence on the effectiveness of the experiments presented. Thirdly, many of the included studies took place in poorly controlled settings or with small sample sizes, which does not allow for generalization of the reported results.
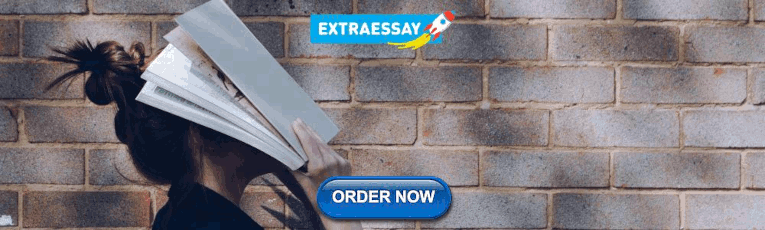
Conclusion and future directions
This study presents a review of the literature recently published in Italian Class A journals on educational robotics. The review conducted suggests, as Benitti ( 2012 ) reported 10 years ago, the limited number of experimental and quantitative studies regarding learning through the use of robotics in education, which would seem to be a general trend in educational research in Italy. Regarding the work on teachers and students' perceptions, questionnaires implemented by the authors and not validated were used (in some cases, only Cronbach's alpha was calculated). This criticality also involves international work, which could be explained by the skills and timing required for statistical validation of an instrument. Interestingly, around 21% of the articles included in this work were written by international academics. This implies a general openness of the journals to research conducted outside national borders and the consequences of the Italian academic community being fully integrated into the international scientific debate. We can also conclude the need to conduct more longitudinal and long-term studies to investigate the impact of ER over time. In fact, only three articles reported experiences longer than 1 year. Two had adults, school teachers and computer science undergraduates as targets ( Cicognini et al., 2019 ; Luccio, 2019 ), and only one investigated a primary school class during the entire 5-year cycle ( Scippo and Ardolino, 2021 ). Most of the studies were conducted in a formal environment, which is in line with other systematic reviews (e.g., Kyriazopoulos et al., 2021 ), indicating a propensity to include robotics practice at an institutional setting. It should be emphasized that, despite general encouragement by institutions and growing interest on the part of teachers, robotics at school has not been systematically integrated into curricula ( Alimisis, 2013 ; Mubin et al., 2013 ; Tzagkaraki et al., 2021 ). ER practice is often characterized as an optional or extracurricular activity. As pointed out by Tzagkaraki et al. ( 2021 ), the analysis of the selected articles in this study does not reveal a link between ER and STEAM (which also sees an interconnection with Art) and there is not enough information on the role of teachers in the implementation of the practice itself. From this systematic research, one can see, in line with Kyriazopoulos et al. ( 2021 ) but in contrast to Benitti ( 2012 ), a decline in the use of LEGO robots in favor of new devices, which are cheaper, in step with technological advances and driven by open-source and maker logic. There is, however, an increase in studies using social robots, although these are very expensive devices. Finally, despite the positive effect of ER attested by the authors on cognitive, social-affective and learning dimension outcomes, there are several cases where the results are not clearly defined and no data were found to support them, thus creating the need for further investigation. Among future prospects, it is interesting to see whether the project proposals included in the review will be realized in quantitative works. Such types of research could enrich the international landscape of empirical research on robotics in education and could be included in a meta-analysis.
Data availability statement
The raw data supporting the conclusions of this article will be made available by the authors, without undue reservation.
Author contributions
The authors have conceived together the overall structure of the paper. The work is the result of a collaborative commitment. Nevertheless section # 1 (Introduction) was edited by LC and AM, section # 2 (Methods) was edited by GB, section # 3 (Results) was edited by SD and LC, and section # 4 (Discussion) was edited by AM. All authors contributed to the article and approved the submitted version.
Conflict of interest
The authors declare that the research was conducted in the absence of any commercial or financial relationships that could be construed as a potential conflict of interest.
Publisher's note
All claims expressed in this article are solely those of the authors and do not necessarily represent those of their affiliated organizations, or those of the publisher, the editors and the reviewers. Any product that may be evaluated in this article, or claim that may be made by its manufacturer, is not guaranteed or endorsed by the publisher.
. ^ 1 Although our research includes works published in Italian scientific journals, it is not intended to take a snapshot of the state of the art of educational research in Italy about educational robotics, but rather to detect the interest in the subject on the part of Italian publishers.
. ^ 2 ANVUR (Italian National Agency for the evaluation of universities and research institutes) makes lists of journals for each scientific field in order to assess academic research. We used history, philosophy and pedagogy (area 11). https://www.anvur.it/en/activities/rating-of-scientific-journals/ .
Alimisis, D. (2013). Educational robotics: open questions and new challenges. Themes Sci. Technol. Educ. 6, 63–71.
Google Scholar
* Alimisis, D., Alimisi, R., and Zoulias, E. (2019). Kids make their own robots: good practices from the eCraft2Learn project. Form@re Open J. Formazione Rete 19, 12–29. doi: 10.13128/formare-24733
CrossRef Full Text | Google Scholar
Anwar, S., Bascou, N. A., Menekse, M., and Kardgar, A. (2019). A systematic review of studies on educational robotics. J. Pre Coll. Eng. Educ. Res. 9, 19–42. doi: 10.7771/2157-9288.1223
* Arar, C., Belazoui, A., and Telli, A. (2021). Adoption of social robots as pedagogical aids for efficient learning of second language vocabulary to children. J. E-Learn. Knowl. Soc. 17, 119–126. doi: 10.20368/1971-8829/1135551
Bacca, J., Baldiris, S., Fabregat, R., Graf, S., and Kinshuk. (2014). Augmented reality trends in education: A systematic review of research and applications. Educ. Techno. Soc. 17, 133–149.
Bagattini, D., and Miotti, B. (2022). Lavorare sul genere a scuola con coding e robotica educativa . Roma: Carocci Editore.
* Balestra, A. (2018). Nuove tecnologie e pensiero computazionale fra passato e presente: un'esperienza didattica nella scuola del primo ciclo. Annali Online Della Didattica e Della Formazione Docente 9, 318–331. doi: 10.15160/2038-1034/1585
Belpaeme, T., Kennedy, J., Ramachandran, A., Scassellati, B., and Tanaka, F. (2018). Social robots for education: a review. Sci. Robot. 3, 1–9. doi: 10.1126/scirobotics.aat5954
PubMed Abstract | CrossRef Full Text | Google Scholar
Benitti, F. B. V. (2012). Exploring the educational potential of robotics in schools: a systematic review. Comput. Educ. 58, 978–988. doi: 10.1016/j.compedu.2011.10.006
Borrego, M., Foster, M. J., and Froyd, J. E. (2015). What is the state of the art of systematic review in engineering education? J. Eng. Educ. 104, 212–242. doi: 10.1002/jee.20069
* Bruni, F., and Nisdeo, M. (2017). Educational robots and children's imagery: a preliminary investigation in the first year of primary school. Res. Educ. Media 9, 37–44. doi: 10.1515/rem-2017-0007
* Campitiello, L., Di Tore, S., Lecce, A., Viola, I., and Sibilio, M. (2021). ASD-ROBOT: an approach that takes into account the sensoriality in the interaction between child, therapist and robot. Italian J. Health Educ. Sports Inclusive Didactics 28, 8–16. doi: 10.17471/2499-4324/1136
* Castro, E., Di Lieto, M., Chiara, P., Inguaggiato, E., Cecchi, F., Dario, P., et al. (2019). Educational Robotics and empowerment of executive cognitive processes: from typical development to special educational needs. Form@re Open J. Formazione Rete 19, 60–77. doi: 10.13128/formare-24782
Ching, Y. H., Yang, D., Wang, S., Baek, Y., Swanson, S., and Chittoori, B. (2019). Elementary school student development of STEM attitudes and perceived learning in a STEM integrated robotics curriculum. TechTrends 63, 590–601. doi: 10.1007/s11528-019-00388-0
* Cicognini, M. E., Miotti, B., and Bizzarri, C. (2019). Educational robotics laboratories for active learning. The case study of Florence schools joining the Le Chiavi della Città project. Form@re Open J. Formazione Rete 19, 149–164. doi: 10.13128/formare-24760
Conti, D., Di Nuovo, S., Buono, S., Trubia, G., and Di Nuovo, A. (2015). “Use of robotics to stimulate imitation in children with Autism Spectrum Disorder: a pilot study in a clinical setting,” in Proceedings of the 24th IEEE International Symposium on Robot and Human Interactive Communication (Kobe).
* Cortiana, P., and Rigotto, C. (2019). Teaching literature through educational robotics: an experience in the primary school. Form@re Open J. Formazione Rete 19, 91–105. doi: 10.13128/formare-24635
Daniela, L., and Lytras, M. D. (2019). Educational robotics for inclusive education. Technol. Knowl. Learn. 24, 219–225. doi: 10.1007/s10758-018-9397-5
* Datteri, E., Bozzi, G., and Zecca, L. (2015). Il “gioco dello scienziato” per l'apprendimento del metodo scientifico nella scuola primaria. TD Tecnologie Didattiche 23, 172–175.
* Datteri, E., and Zecca, L. (2017). Theoretical vocabularies and styles of explanation of robot behaviours in children. Res. Educ. Media 9, 3–9. doi: 10.1515/rem-2017-0002
* De Carolis, B., Palestra, G., Della Penna, C., Cianciotta, M., and Cervelione, A. (2019). Social robots supporting the inclusion of unaccompanied migrant children: teaching the meaning of culture-related gestures. J. E-Learning Knowl. Soc. 15, 43–57. doi: 10.20368/1971-8829/1636
* Di Tore, S., Todino, M. D., and Sibilio, M. (2019). Disuffo: Design, prototyping and development of an open-source educational robot. Form@re Open J. Formazione Rete 19, 106–116. doi: 10.13128/formare-24446
Dunst, C. J., Trivette, C. M., Prior, J., Hamby, D. W., and Embler, D. (2013). Parents' judgments of the acceptability and importance of socially interactive robots for intervening with young children with disabilities. Soc. Robots Res. Rep. 1, 1–5.
Ferrari, E., Robins, B., and Dautenhahn, K. (2009). “Therapeutic and educational objectives in robot assisted play for children with autism,” in The 18th IEEE International Symposium on Robot and Human Interactive Communication (Toyama).
* Ferrari, L., Macauda, A., Soriani, A., and Russo, V. (2020). Educational robotics and artificial intelligence education: what priorities for schools? TT - Robotica educativa ed educazione all'intelligenza artificiale: quali priorità per la scuola? Form@re Open J. Formazione Rete 20, 68–85. doi: 10.13128/form-10038
Frison, D. (2019). Educational robotics in the early childhood settings 0-6: a systematic review. Form@re Open J. Formazione Rete 19, 30–46. doi: 10.13128/formare-24937
* Gasparini, S. (2019). Social robots and L2 teaching for children: a preliminary study on the embodiment of gestures Robot sociali e insegnamento di L2 ai bambini: uno studio preliminare sull'embodiment della gestualità. Form@re Open J. Formazione Rete , 19, 47–59. doi: 10.13128/formare-24600
* Giannandrea, L., Gratani, F., and Renieri, A. (2020). Crossing boundaries: documentation of a teacher training course on design, robotics and coding. Res. Educ. Media 12, 85–92. doi: 10.2478/rem-2020-0010
* Gramigna, A. (2018). RoboticaMente: metacognizione e innovazione nella scuola dei talenti RoboticaAlly: metacognition and innovation in talent's school. Formazione Insegnamento 16, 291–304. doi: 10.107346/-fei-XVI-02-18_23
Jung, S. E., and Won, E. S. (2018). Systematic review of research trends in robotics education for young children. Sustainability 10, 1–24. doi: 10.3390/su10040905
Karim, M. E., Lemaignan, S., and Mondada, F. (2015). “A review: can robots reshape K-12 STEM education?” in Proceedings of IEEE Workshop on Advanced Robotics and Its Social Impacts, ARSO . doi: 10.1109/ARSO.2015.7428217
Kyriazopoulos, I., Koutromanos, G., Voudouri, A., and Galani, A. (2021). “Educational robotics in primary education: A systematic literature review,” in Handbook of Research on Using Education Robotics to Facilitate Student Learning , eds St. Papadakis and M. Kalogiannakis (IGI Global),377–401. doi: 10.4018/978-1-7998-6717-3.ch015
* Lehmann, H., and Svarny, P. (2021). Using a social robot for different types of feedback during university lectures. Educ. Sci. Soc. 2, 282–295. doi: 10.3280/ess2-2021oa12485
Leite, I., Martinho, C., and Paiva, A. (2013). Social robots for long-term interaction: a survey. Int. J. Soc. Robot. 5, 291–308. doi: 10.1007/s12369-013-0178-y
* Luccio, F. L. (2019). Learning distributed algorithms by programming robots. J. E-Learn. Knowl. Soc. 15, 89–100. doi: 10.20368/1971-8829/1625
Moher, D., Liberati, A., Tetzlaff, J., and Altman, D. G. (2009). Preferred reporting items for systematic reviews and meta-analyses: The PRISMA statement. PLoS Med. 6, e1000097. doi: 10.1371/journal.pmed.1000097
* Mortari, L., Silva, R., and Zanotti, A. (2020). Quando il Service Learning pone la ricerca educativa a servizio della formazione docente e dell'innovazione didattica: il “caso” Resolving Robots. RicercAzione 12, 83–105. doi: 10.32076/RA12106
Mubin, O., Stevens, C. J., Shahid, S., Mahmud, A., Al, and Dong, J.-J. (2013). A review of the applicability of robots in education. Technol. Educ. Learn. 1, 1–7. doi: 10.2316/Journal.209.2013.1.209-0015
* Negrini, L. (2020). Teachers' attitudes towards educational robotics in compulsory school. Gli atteggiamenti degli insegnanti della scuola dell'obbligo nei confronti della robotica educativa. TD Tecnologie Didattiche 77–90.
* Negrini, L., and Giang, C. (2019). How do pupils perceive educational robotics as a tool to improve their 21st century skills? J. E-Learning Knowl. Soc. 15, 77–87. doi: 10.20368/1971-8829/1628
Papadakis, S., and Kalogiannakis, M. (2022). “Exploring preservice teachers' attitudes about the usage of educational robotics in preschool education,” in Research Anthology on Computational Thinking, Programming, and Robotics in the Classroom (IGI Global), 807–823.
Papadakis, S., Vaiopoulou, J., Sifaki, E., Stamovlasis, D., and Kalogiannakis, M. (2021). Attitudes towards the use of educational robotics: exploring pre-service and in-service early childhood teacher profiles. Educ. Sci. 11, 204. doi: 10.3390/educsci11050204
Papadopoulos, I., Lazzarino, R., Miah, S., Weaver, T., Thomas, B., and Koulouglioti, C. (2020). A systematic review of the literature regarding socially assistive robots in pre-tertiary education. Comput. Educ. 155, 103924. doi: 10.1016/j.compedu.2020.103924
Papert, S. (1980). Mindstorms-Children, Computers and Powerful Ideas . New York, NY: Basic Books.
* Passalacqua, F., and Zecca, L. (2019). Valutare laboratori di robotica educativa: studio di un approccio partecipativo evaluating educational robotics laboratories: a study of a participatory approach. Formazione Insegnamento 17, 449–456. doi: 10.107346/-fei-XVII-01-19_35
* Pennazio, V. (2017). Social robotics to help children with autism in their interactions through imitation. Res. Educ. Media 9, 10–16. doi: 10.1515/rem-2017-0003
Pivetti, M., Di Battista, S., Agatolio, F., Simaku, B., Moro, M., and Menegatti, E. (2020). Educational Robotics for children with neurodevelopmental disorders: a systematic review. Heliyon 6, e05160. doi: 10.1016/j.heliyon.2020.e05160
* Rubinacci, F., Ponticorvo, M., Passariello, R., and Miglino, O. (2017). Robotics for soft skills training. Res. Educ. Media 9, 20–25. doi: 10.1515/rem-2017-0010
* Scippo, S., and Ardolino, F. (2021). Computational thinking in Montessori primary school. Ricerche Di Pedagogia e Didattica 2, 59–76. doi: 10.6092/issn.1970-2221/12163
Spolaôr, N., and Benitti, F. B. V. (2017). Robotics applications grounded in learning theories on tertiary education: a systematic review. Comput. Educ. 112, 97–107. doi: 10.1016/j.compedu.2017.05.001
Sullivan, A., and Bers, M. U. (2019). Investigating the use of robotics to increase girls' interest in engineering during early elementary school. Int. J. Technol. Design Educ. 29, 1033–1051. doi: 10.1007/s10798-018-9483-y
Toh, L. P. E., Causo, A., Tzuo, P. W., Chen, I. M., and Yeo, S. H. (2016). A review on the use of robots in education and young children. Educ. Technol. Soc. 19, 148–163.
* Torre, M. (2019). Seven Ozobot messengers: a story by Buzzati between mathematics and robotics I sette messaggeri Ozobot: un racconto di Buzzati tra matematica e robotica. Form@re Open J. Formazione Rete 19, 392–400. doi: 10.13128/formare-24519
Tzagkaraki, E., Papadakis, S., and Kalogiannakis, M. (2021). “Exploring the use of educational robotics in primary school and its possible place in the curricula,” in Educational Robotics International Conference EDUROBOTICS 2021 , Vol. 982, ed M. Malvezzi (Springer International Publishing),216–229.
* Van Den Heuvel, R., Lexis, M., and De Witte, L. (2016). Giochi di prossima generazione I robot per supportare il gioco dei bambini con severe disabilità fisiche. L'integrazione Scolastica e Sociale 15, 125–138.
Xia, L., and Zhong, B. (2018). A systematic review on teaching and learning robotics content knowledge in K-12. Comput. Educ. 127, 267–282. doi: 10.1016/j.compedu.2018.09.007
* Zannini, L., Mazzolatti, D., Bernardelli, G., Antonacci, F., Borghese, N., and Daniele, K. (2019). Evaluating online games/activities by a group of elderly in a robotic experience aimed at supporting their independent living. Form@re Open J. Formazione Rete 19, 312–327. doi: 10.13128/formare-24615
* ^ Studies included in the Systematic Review.
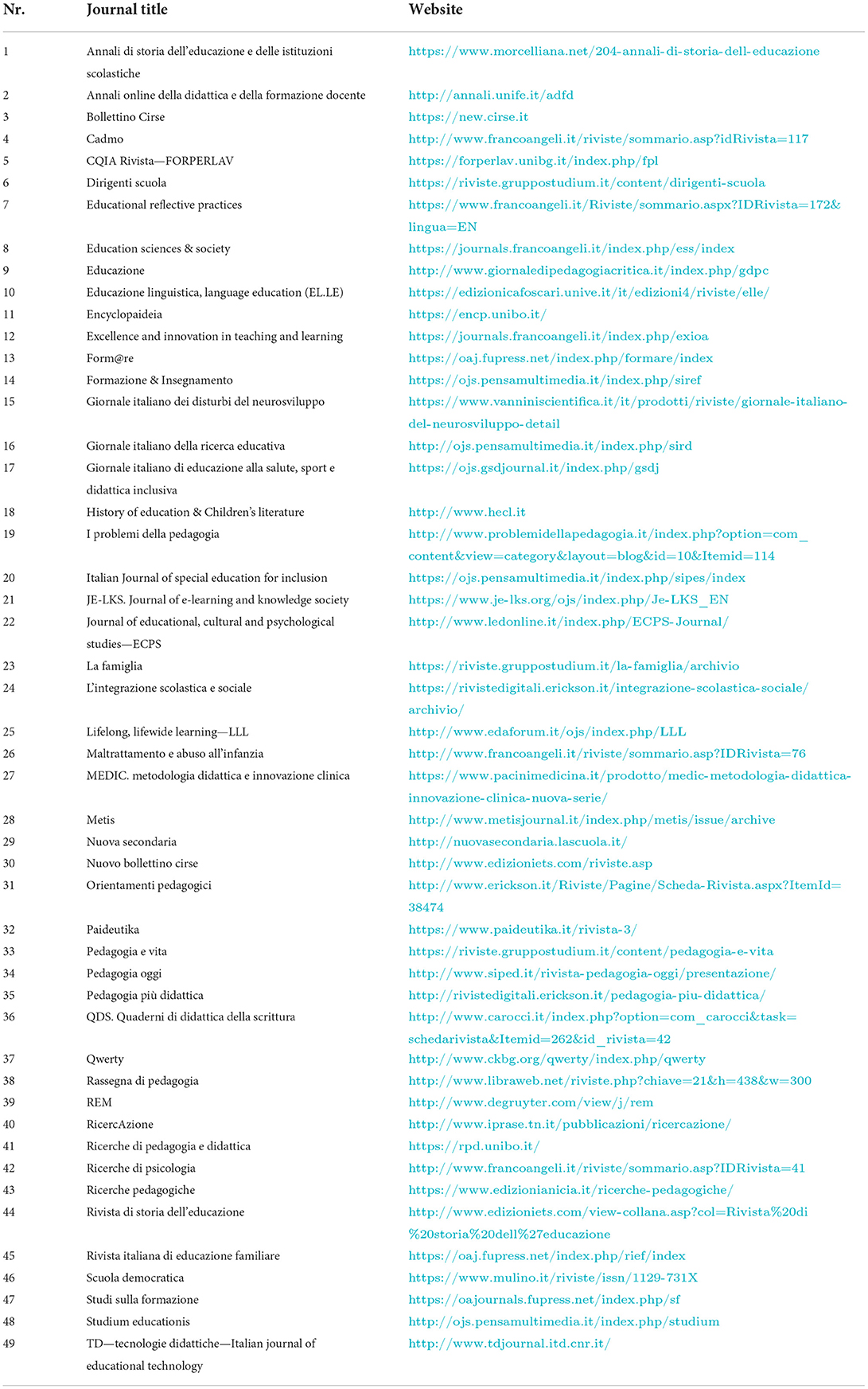
Table A1 . Class A Italian journals.
Keywords: educational robotics, Italian journals, social robotics, systematic review, STEM
Citation: Bonaiuti G, Campitiello L, Di Tore S and Marras A (2022) Educational robotics studies in Italian scientific journals: A systematic review. Front. Educ. 7:1005669. doi: 10.3389/feduc.2022.1005669
Received: 28 July 2022; Accepted: 21 September 2022; Published: 13 October 2022.
Reviewed by:
Copyright © 2022 Bonaiuti, Campitiello, Di Tore and Marras. This is an open-access article distributed under the terms of the Creative Commons Attribution License (CC BY) . The use, distribution or reproduction in other forums is permitted, provided the original author(s) and the copyright owner(s) are credited and that the original publication in this journal is cited, in accordance with accepted academic practice. No use, distribution or reproduction is permitted which does not comply with these terms.
*Correspondence: Giovanni Bonaiuti, giovanni.bonaiuti@unica.it
This article is part of the Research Topic
Educational Robotics as a Tool to Foster 21st Century Skills
- Open access
- Published: 21 March 2024
Accuracy of implant placement with computer-aided static, dynamic, and robot-assisted surgery: a systematic review and meta-analysis of clinical trials
- Angkoon Khaohoen 1 ,
- Warit Powcharoen 2 ,
- Tanapon Sornsuwan 3 ,
- Pisaisit Chaijareenont 1 ,
- Chaiy Rungsiyakull 4 &
- Pimduen Rungsiyakull 1
BMC Oral Health volume 24 , Article number: 359 ( 2024 ) Cite this article
261 Accesses
Metrics details
This systematic review explores the accuracy of computerized guided implant placement including computer-aided static, dynamic, and robot-assisted surgery. An electronic search up to February 28, 2023, was conducted using the PubMed, Embase, and Scopus databases using the search terms “surgery”, “computer-assisted”, “dynamic computer-assisted”, “robotic surgical procedures”, and “dental implants”. The outcome variables were discrepancies including the implant’s 3D-coronal, -apical and -angular deviations. Articles were selectively retrieved according to the inclusion and exclusion criteria, and the data were quantitatively meta-analysed to verify the study outcomes. Sixty-seven articles were finally identified and included for analysis. The accuracy comparison revealed an overall mean deviation at the entry point of 1.11 mm (95% CI: 1.02–1.19), and 1.40 mm (95% CI: 1.31–1.49) at the apex, and the angulation was 3.51˚ (95% CI: 3.27–3.75). Amongst computerized guided implant placements, the robotic system tended to show the lowest deviation (0.81 mm in coronal deviation, 0.77 mm in apical deviation, and 1.71˚ in angular deviation). No significant differences were found between the arch type and flap operation in cases of dynamic navigation. The fully-guided protocol demonstrated a significantly higher level of accuracy compared to the pilot-guided protocol, but did not show any significant difference when compared to the partially guided protocol. The use of computerized technology clinically affirms that operators can accurately place implants in three directions. Several studies agree that a fully guided protocol is the gold standard in clinical practice.
Peer Review reports
Currently, implants to replace missing teeth play a significant role in dental treatment because of their exceptional survival rate in restoring both partial and complete edentulism [ 1 , 2 ]. To improve the long-term success rate of final implant prostheses, prosthetically-driven implant placement with computerized implant technology is the most crucial factor in overcoming a placement’s early or late failure [ 3 , 4 ].
Cone-beam computed tomography (CBCT) is used in implant planning to generate multiplanar and three-dimensional pictures that enhance the clinical outcome. By incorporating CBCT into implant planning, clinicians can evaluate the condition of the alveolar bone, which is directly related to the success rate of implants, in order to predict primary stability [ 5 ]. This information can assist the surgeon in choosing suitable dental implant and surgical techniques prior to careful placement. In addition, CBCT combined with digital scanning images can be transferred to specific software and utilized to anticipate virtual crowns before the intraoperative procedure [ 6 ].
Recently, computerized guided implant placement has been widely used to assist operators in reducing the unexpectedly deviated position and unfortunate results after osteotomy. This system can be divided into three subgroups: static, dynamic computer-aided, and robot-assisted implant surgery [ 7 ]. Static computer-aided implant surgery (sCAIS) systems are digitally programmed fabricated surgical templates designed to represent the final implant’s position [ 8 ]. Dynamic CAIS (dCAIS) provides a real-time procedure related to the dental drill using optical or mechanical tracking technology without a template guide co-operated through a nearby monitor [ 9 ]. Nevertheless, d-CAIS is devoid of physical guidance and has an associative learning curve [ 10 ]. Simultaneously, robotic CAIS (rCAIS) combines the benefits of avoiding the physical constraints of s-CAIS, the instantaneous feedback of d-CAIS, and the accurate control achieved by robotic arms [ 11 ]. Robot-assisted implant systems have been developed to improve precision and accuracy, lessen human-based errors, and eliminate the use of static guides [ 12 ]. Yomi [ 11 ] is the first robotic implant surgical system approved by the Food and Drug Administration (FDA). It is known as a semi-active robot assistance system since the surgeon can manually conduct the implant osteotomy through a robotic arm. Recently, China’s National Medical Product Administration authorized Remebot, an autonomous robot-assisted surgery system. In addition to being a semi-active device, it is also a task-autonomous robotic system that can drill and place the implant autonomously in the planned position [ 13 ].
Even though digital implant technology assists in reducing the errors cause by operator- or patient-related factors, the accumulative errors in computer-guided systems still exist as the result of preoperative or intraoperative procedures or postoperative evaluation [ 9 ], as shown in Table 1 . The flaws of navigation systems have been clinically reported. For instance, a common weak point in a static surgical guide is the manufacturing error, which can hamper the proper positioning and stability of the guide during the operation [ 8 , 14 ]. The occurrence of errors in guide systems can be influenced by several factors, including the quality of the acquired image and the expertise and understanding of the system's operator. Dynamic navigation, on the contrary, can guide the osteotomy and implant without template guidance. Some authors claim that the learning curve is influenced by the operator’s personal confidence with the computer and the 3D system [ 15 , 16 ]. However, this system appears to provide more advantages for novice professionals by minimizing their mistakes. Put simply, it has the potential to enhance the proficiency of novice surgeons. Although robot-assisted dental surgery provides haptic and visual guidance during implant surgery [ 11 ], the sum of errors can derive from the complexity of multiple Digital Imaging and Communications in Medicine (DICOM) files and the instability of polymethyl methacrylate (PMMA) guide fixation. The drawback to using multiple superimposition phases involves the utilization of various DICOM files or CBCT scans, including the preoperative and intraoperative scans. Each instance of superimposition has the potential to lead to mistakes. Hence, it is imperative to meticulously verify every step of the merging process for correctness. Furthermore, the attachment of PMMA guides presents a challenging scenario. Unstable occlusal splint guidance might lead to inaccurate implant placement. In instances such as those involving movable teeth, large crowns and bridges, or many dental implants, an edentulous splint can be utilized instead of a dentate splint. There are individuals who have an allergy to PMMA. Two potential options for splinting are the new dentate splint or the clamp splint [ 7 , 17 ]. The advantages and disadvantages of robotic systems compared to static and dynamic systems are summarized in Table 2 .
Several previous systematic reviews have summarized the accuracy of static and dynamic computer-assisted systems and the factors relating to their accuracy. The findings indicate that the utilization of d-CAIS enhanced the accuracy of implant placement in comparison to the freehand technique, and also modestly reduced the angular deviation when compared to s-CAIS [ 10 ]. In addition, it provided a safety range that is considered appropriate for clinical use [ 9 ]. Nevertheless, there have been inadequate studies investigating the use of robot-assisted implant systems in clinical trials, and no systematic analysis has been conducted to compare the static, dynamic, and robot-assisted systems.
Regarding static systems, Tahmaseb, et.al . [ 29 ] found that partial edentulism is often more favorable than complete edentulism, except in angular deviation. Zhou and colleagues [ 20 ] concluded that a fully guided protocol, which involves employing a fixation screw and a flapless technique, showed the most remarkable accuracy. Furthermore, they stated that there was a statistically significant difference in angular deviation, favoring the mandibular arch over the maxillary arch. In cases of dynamic systems, Wei and colleagues [ 9 ] evaluated the accuracy of d-CAIS and identified the factors that influence its accuracy. However, the impact of significant clinical factors on the accuracy of the navigation system is obsolete, and some clinical aspects have not been assessed, such as the lack of comparisons regarding the surgical protocol in the static system or the arch and flap techniques in the dynamic system.
The key difference in this systematic review, in comparison to others, is the separate evaluation of robot guided surgery and its comparison to other subgroups. Therefore, this systematic review aims to explore the clinical accuracy of computerized guided surgery for dental implant placement based on a virtual plan and later aims to identify the relevant factors affecting its accuracy.
Our review was enrolled in the International Prospective Register of Systematic Reviews (PROSPERO); the registered protocol number is CRD42022332900. This systematic review performed documentation management following the Preferred Reporting Item for Systematic Reviews and Meta-analysis (PRISMA) guidelines.
PICO question
Based on the PRISMA guidelines, our systematic review illustrated the accuracy of implant placement using computer-aided static, dynamic, and robot-assisted surgery. The cornerstone question was: “What is the accuracy of a computer-guided implant system in relation to the preoperative plan?”.
Search strategy
The formulation of a research question following the PICO methodology was used to define the scope. An electronic and manual search of PubMed, Embase, and Scopus revealed limited sources with the identified relevant keywords included in the title, abstract, and subject terms.
An initial search was performed with the terms, as shown in Table 3 [ 9 , 10 ]. Papers published in the last thirteen years (between January 1, 2010 and February 28, 2023) in the English language and indexed in the aforementioned databases were searched.
Study selection
Two reviewers (K.A. and R.P.) screened all abstracts and titles independently. In the event of disagreements or arguments, the discussion was solved by consensus or a third opinion (C.P.). No kappa score was computed. The reasons for the exclusion of the abstracts and titles that were not further included in this review were that (a) they comprised review literature and preclinical studies, (b) they were on unrelated topic and dental implants, or (c) the parameters were unclear. The articles retrieved from the search and the inclusion and exclusion criteria were obtained for data synthesis. For evaluation, the full-text criteria are listed in Table 4 .
Data extraction
After the first author (K.A.) and second author (R.P) independently selected and retrieved articles against the inclusion criteria, the data from the articles were collected and organized, and the extracted data were recorded in an Excel spreadsheet (Microsoft Corporation, Redmond, WA, USA). The data were grouped to analyze the following variables as shown in Tables 5 , 6 , 7 and 8 . Each system was separated into static, dynamic, and robotic groups to evaluate the discrepancies: deviation at the entry point and the apex in a three-dimensional direction and deviation of the axis (Fig. 1 ). The data on the flap operation (flapless or open flap) and different jaw bones involved in implant placement (maxilla or mandible) in dynamic navigation systems were recorded. Additionally, static systems were analyzed regarding whether the surgical protocol was a pilot or partial or full protocol.
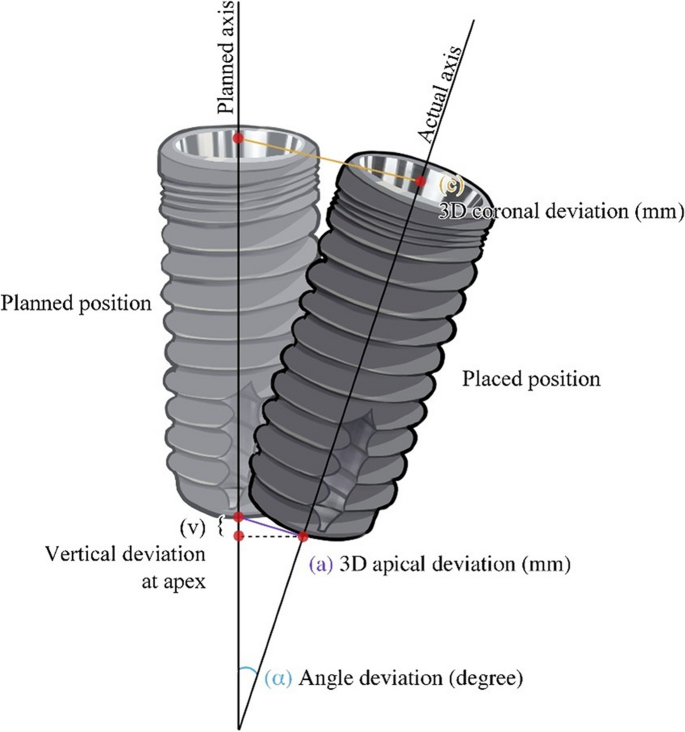
Illustrates the analytic parameters of the accuracy of dental implant navigation systems in this review study. Global angular (α) is the 3D angle between the central axis of the planned and the placed position. Global coronal (c) is the 3D distances between the coronal centers of the planned and actual position. Global apical (a) is the 3D distances between the apical centers of the planned and actual position
Quality assessment
The quality of the included RCTs was evaluated using the Cochrane risk of bias (RoB-2) tool [ 84 ]. Relying on the descriptions given for each criterion, a scoring of low concern, some concern, or a high risk of bias was assigned. At the same time, the Robins-I was used to evaluate the risk of bias in the non-randomized clinical trials that were included [ 85 ]. The rating of each criterion was the same using RCT. Furthermore, four case studies were analyzed using JBI checklist [ 86 ].
Statistical analysis
Statistical analysis was performed using RevMan 5 (Review manager version 5; The Cochrane Collaboration, Copenhagen, Denmark) and Microsoft Excel: Meta-Essentials workbooks for meta-analysis version 1.5 (Suurmond R et al., 2017) [ 87 ]. The overall accuracy of computerized guided implant placement and selected influential factors were evaluated. Due to the heterogeneity between the articles, totals were evaluated using random-effects models for continuous variables. Three parameters (coronal, apical, and angular deviations) were analyzed separately. Additionally, forest plots were used to estimate the overall results from the mean and standard deviation (SD) weighted by the size of each group and the means of the meta-analysis had a corresponding 95% confidence interval. The significance level of the tests was 0.05 [ 88 ].
The navigation system group is reported in the descriptive analysis. Pairwise meta-analyses were performed to evaluate the factors of flap operation, arch type, and guided surgery protocol wherein the mean differences were evaluated using random-effect models.
Heterogeneity and publication bias
The Q heterogeneity statistic and corresponding P value for the chi-squared test were analyzed. A P value of the Q statistic of < 0.05 was considered statistically significant. The percentage of the variability (I 2 ) values of ≥ 25%, 50%, and 75% corresponded to the cut-off points for low, moderate, and high degrees of heterogeneity, respectively.
Data selection
According to the modified PRISMA 2020 diagram (Fig. 2 ), the initial electronic and manual search for studies through PubMed, Scopus, and Embase identified 1,115 articles. After the exclusion of duplicated articles, 515 articles were available for screening. Three hundred and seventy-five references were excluded after title and abstract screening. One hundred and forty lists were appraised for independent full-text reviewers. The inclusion and exclusion criteria were applied, and sixty-seven articles met the inclusion criteria and qualitative synthesis. The studies selected from the inclusion criteria are summarized in Table 5 , 6 , 7 and 8 .
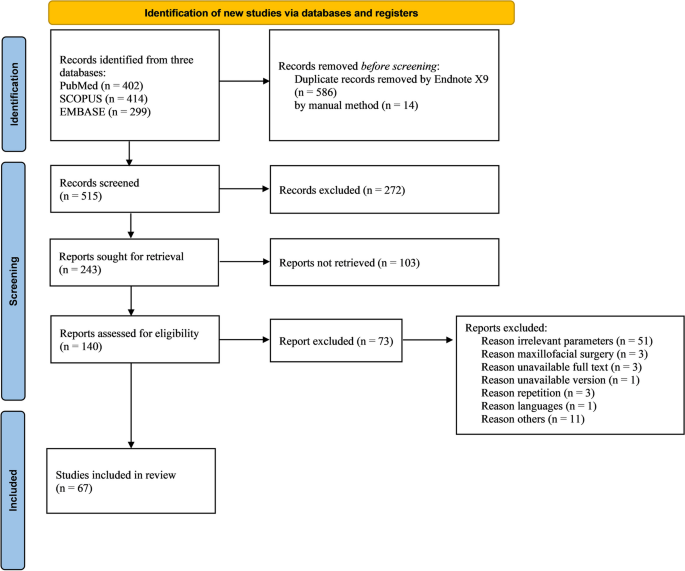
Modified PRISMA 2020 flow diagram of the process and results of literature search
Study characteristics
A total of 67 studies were included in this study. Of the 67 studies, 19 reported randomized clinical studies, 23 reported prospective studies, 21 reported retrospective studies, and 4 reported case studies. According to navigation systems (5,673 implants), 53 studies (4,504 implants) assessed the outcomes of static guided surgery, 15 studies (1,125 implants) reviewed the outcomes of dynamic guided surgery, and 2 studies (44 implants) analyzed the outcomes of robot-assisted surgery.
Comparisons between pre- and post- dental implant placements can be found among the three navigation types, namely, 43 studies on static systems [ 8 , 18 , 19 , 21 , 22 , 30 , 31 , 32 , 33 , 34 , 35 , 36 , 37 , 38 , 39 , 40 , 41 , 42 , 43 , 44 , 45 , 46 , 47 , 48 , 49 , 50 , 51 , 52 , 53 , 54 , 55 , 56 , 57 , 58 , 59 , 60 , 61 , 62 , 63 , 64 , 65 , 66 , 67 ], 7 studies on dynamic systems [ 7 , 16 , 25 , 68 , 69 , 70 , 71 ], and only 2 on robot-assisted surgery [ 11 , 13 ]. Nevertheless, 15 studies [ 12 , 15 , 26 , 72 , 73 , 74 , 75 , 76 , 77 , 78 , 79 , 80 , 81 , 82 , 83 ] showed comparative data between systems. To be more precise, seven studies assessed the accuracy between freehand and static navigation, three studies compared freehand and dynamic navigation, three studies compared static and dynamic systems, and two studies reported the difference between freehand, static, and dynamic systems.
Quality of evidence
The bias risk analysis for the clinical studies that we included is summarized in Fig. 3 and Fig. 4 . Overall, the nineteen RCTs [ 12 , 22 , 26 , 42 , 54 , 59 , 64 , 65 , 66 , 69 , 72 , 74 , 75 , 76 , 77 , 79 , 80 , 81 , 82 ] were assessed using RoB-2. Nine presented a “low risk” of bias, while eight presented “some concerns”. Two studies were identified as having a “high risk” of bias. Six RCTs showed “some concerns” regarding the outcome measurements because of their article’s awareness of outcome assessors. Meanwhile, one study was of “high risk” of bias. For “deviations from the intended interventions”, three studies exhibited “some concern”. Additionally, one study was of “some concern” and one study was of “high risk” in the randomization process.
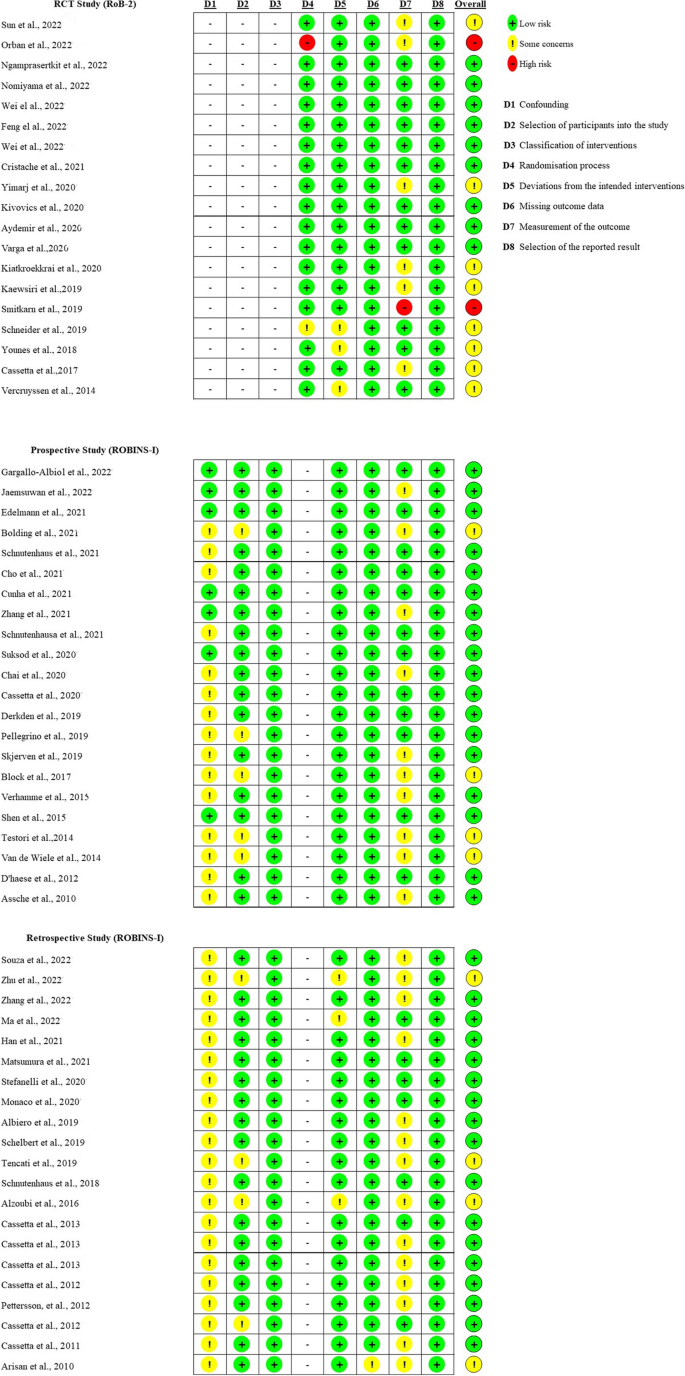
Summary of the bias risk assessment of the randomized controlled trial, prospective, and retrospective studies
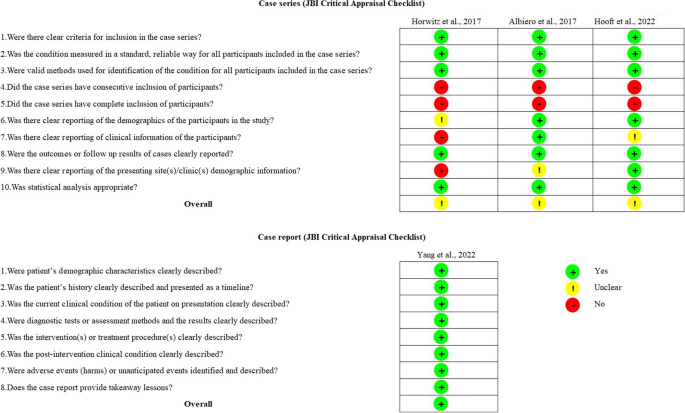
Summary of the bias risk assessment of the case studies
Four articles showed “some concern” among selected prospective clinical studies [ 8 , 11 , 15 , 16 , 18 , 21 , 30 , 38 , 39 , 40 , 46 , 49 , 50 , 52 , 53 , 55 , 56 , 57 , 58 , 61 , 68 , 73 , 83 ]. Overall, the eighteen articles appeared to be high-quality assessments. For the “confounding” factor, only eight articles were associated with a “low risk”, while fifteen were found to raise “some concerns” due to being uncontrolled for the critical or time-varying confounding domains. For the “measurement of the outcome” risk category, ten studies were unclear about the blinded assessor or used a non-blinded examiner. Meanwhile, twelve studies were classified as having a “low risk” of bias. Notably, “selection of participants into the study” was related to intervention and outcome, which was called selection bias. There were only five studies that exhibited “some concerns”. Additionally, there were classifications of “low risk” for all studies in “classification of the interventions”, “deviation from the intended interventions”, “missing outcome data”, and “selection of the reported result”.
Similar to the prospective study design, a total of twenty-one retrospective non-RCTs [ 7 , 19 , 25 , 31 , 32 , 33 , 34 , 35 , 36 , 37 , 41 , 45 , 47 , 48 , 51 , 60 , 62 , 63 , 67 , 70 , 78 ] were assessed using Robin-I. According to Fig. 3 , concerns about “confounding” bias were referred to in all studies. For the outcome measurement, fourteen presented “some concerns”, while seven were classified as having a “low risk” of bias. Additionally, four studies were found to have “some concerns” when selecting participants. Similarly, there were three concerns in “deviations from intended interventions” and only one concern in “missing outcome data”. Overall, seventeen retrospective studies were “low risk”, while four were identified as showing “some concerns”.
According to Fig. 4 , three case series [ 43 , 44 , 71 ] and one case report [ 13 ] were assessed using JBI Critical Appraisal Checklist. Conclusively, all case series were classified as having an “unclear” assessment, while only one case report seemed to be a high-quality assessment.
Accuracy outcomes
Overall computerized guided implant placement.
Regarding global deviation at both the coronal and apical sections, and angular deviation in the implants, 67 studies (n = 5,673 implants) were divided into 53 static, 15 dynamic, and 2 robot-assisted surgeries. At the level of the implant shoulder, the overall weighted mean coronal deviation was 1.11 mm (95% CI: 1.02–1.19 mm; Fig. 5 ). At the level of the implant apex, the weighted mean apical deviation was 1.40 mm (95% CI: 1.31–1.49; Fig. 6 ). The overall weighted mean angular deviation was 3.51˚ with a 95% CI of 3.27˚-3.75˚ (Fig. 7 ).
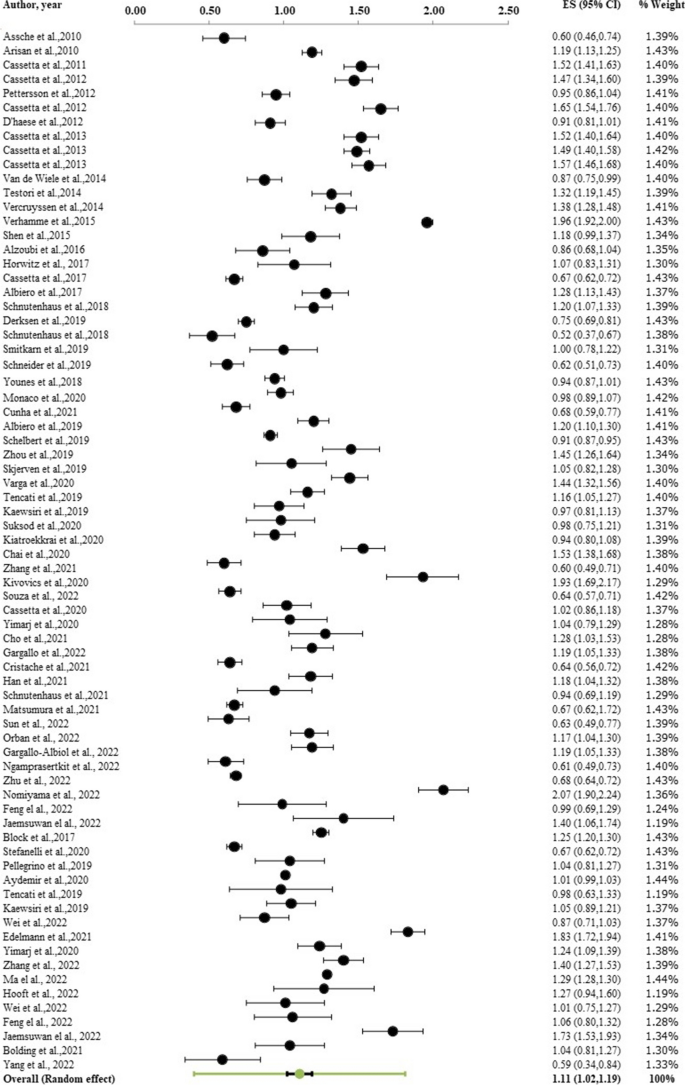
Forest plot showing global deviation (coronal) of computerized guided implant placement (95% CI)
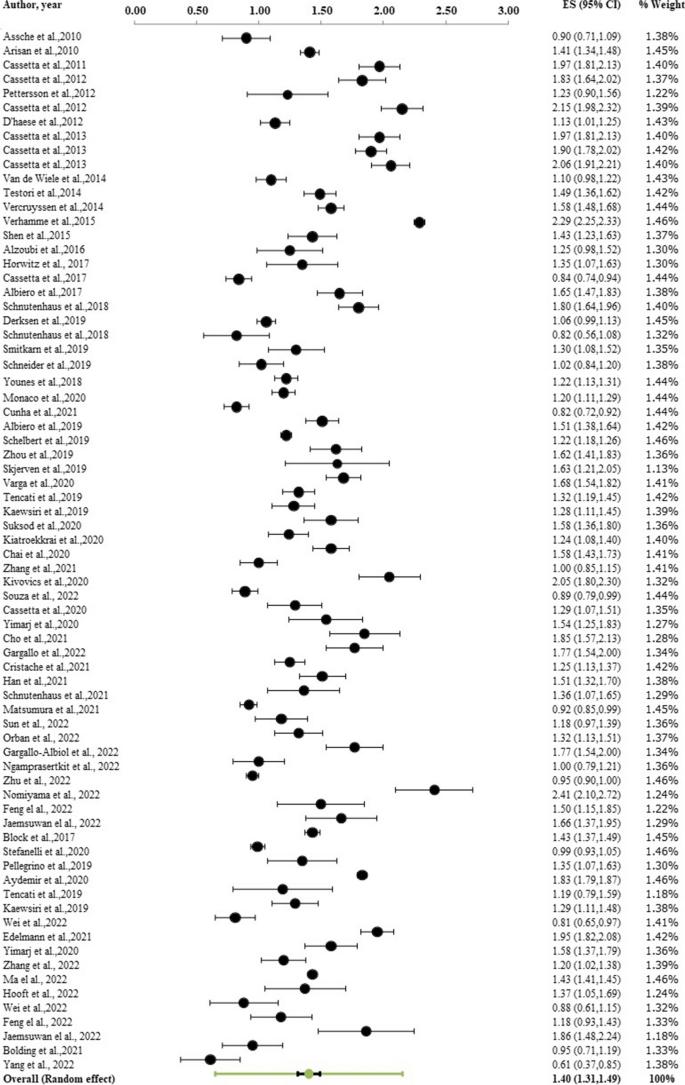
Forest plot showing global deviation (apical) of computerized guided implant placement (95% CI)
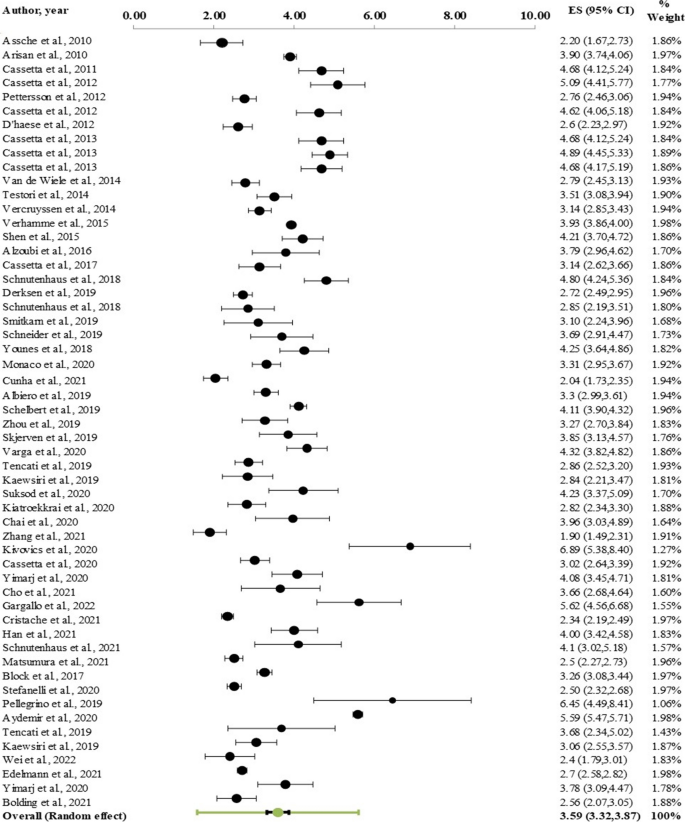
Forest plot showing angular deviation of computerized guided implant placement (95% CI)
Subgroup analysis: navigation system
The static, dynamic, and robotic approaches displayed a mean deviation at the entry point of 1.11 mm (95% CI: 1.00–1.20), 1.18 (95% CI: 1.02–1.34), and 0.81 (95% CI: 0.37–1.25), respectively (Fig. 8 ). At the apical point, the overall weighted mean deviation was 1.44 mm (95% CI: 1.34–1.54) in the static system, 1.36 (95% CI: 1.18–1.54) in the dynamic system, and 0.77 (95% CI: 0.43–1.11) in robot-assisted surgery (Fig. 9 ). For the angular deviation, the overall weighted mean deviation of these systems was 3.58˚ (95% CI: 3.33–3.83), 3.51˚ (95% CI: 2.90–4.12), and 1.71˚ (95% CI: 0.04–3.38), respectively (Fig. 10 ).

Forest plot showing global deviation (coronal) of static-, dynamic-, and robotic-guided surgery (95% CI)

Forest plot showing global deviation (apical) of static-, dynamic-, and robotic-guided surgery (95% CI)

Forest plot showing angular deviation of static-, dynamic-, and robotic-guided surgery (95% CI)
Effect of the arch type on the dynamic navigation system (maxillary or mandibular arch)
One prospective [ 68 ] and one retrospective study [ 25 ] ( n = 156 implants) were reviewed to compare the accuracy of dynamic navigation surgery performed on the maxillary or mandibular arch. The meta-analysis data showed no statistically significant differences in global coronal deviation (MD:0.06 mm; 95% CI: -0.03 to 0.16; P ≥ 0.001; I 2 = 0%), global apical deviation (MD:0.14 mm; 95% CI: 0.03 to 0.24; P ≥ 0.001; I 2 = 0%), or angular deviation (MD:0.38 mm; 95% CI: -0.26 to 1.02; P ≥ 0.001; I 2 = 72%) when comparing the maxillary and mandibular arches. Coronal and apical deviations exhibited a low degree of homogeneity between the studies. In comparison, angular deviation showed a high degree of heterogeneity between the studies (Fig. 11 ).
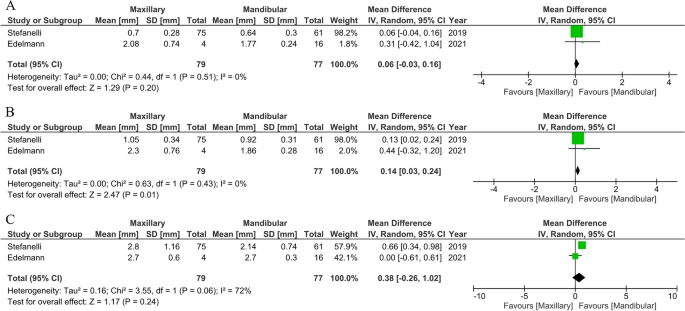
Forest plots of global coronal ( A ), global apical ( B ), and angular deviation ( C ) comparing clinical study of dynamic system in different arches
Effect of the flap operation on the dynamic navigation system (flapless and open-flap)
Only two articles [ 16 , 68 ] (prospective studies; n = 38) were evaluated to compare the accuracy of dynamic navigation surgery performed using flapless and open-flap procedures. Two studies reporting data in a meta-analysis described no statistically significant differences in global coronal deviation (MD:-0.11 mm; 95% CI: -0.36 to 0.13; P ≥ 0.001; I 2 = 0%), global apical deviation (MD:-0.03 mm; 95% CI: -0.32 to 0.26; P ≥ 0.001; I 2 = 0%), and angular deviation (MD:0.65 mm; 95% CI: -0.73 to 2.02; P ≥ 0.001; I 2 = 20%) (Fig. 12 ). No heterogeneity was observed between the studies on coronal and apical deviations. A low degree of homogeneity between the studies was found in the angular deviation.
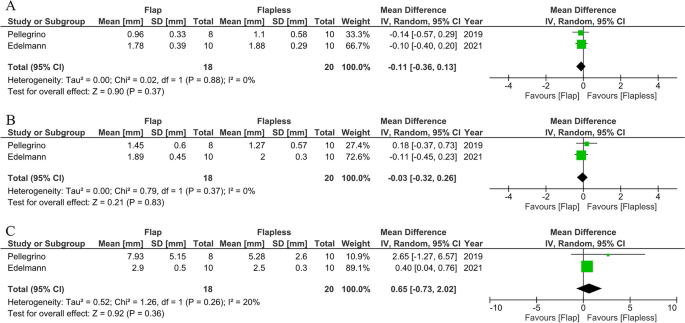
Forest plots of global coronal ( A ), global apical ( B ), and angular deviation ( C ) comparing clinical study of the dynamic system between the flap and flapless protocols
Effect of the surgical protocol for a static navigation system (pilot, partial, or full protocol)
Two RCT studies [ 76 , 77 ] ( n = 146 implants) were reported to compare the accuracy of static navigation systems performed using pilot and fully guided protocols. The MD meta-analysis reported statistically significant differences favoring the full protocol in global coronal deviation (MD:0.33 mm; 95% CI: 0.14 to 0.52; P < 0.001; I 2 = 52%), global apical deviation (MD:0.44 mm; 95% CI: 0.32 to 0.56; P < 0.001; I 2 = 6%), and angular deviation (MD:3.29 mm; 95% CI: 2.36 to 4.21; P < 0.001; I 2 = 59%). A medium-to-high heterogeneity between the studies was found for coronal and angular deviations. However, a low degree of homogeneity was found for apical deviation (Fig. 13 ).
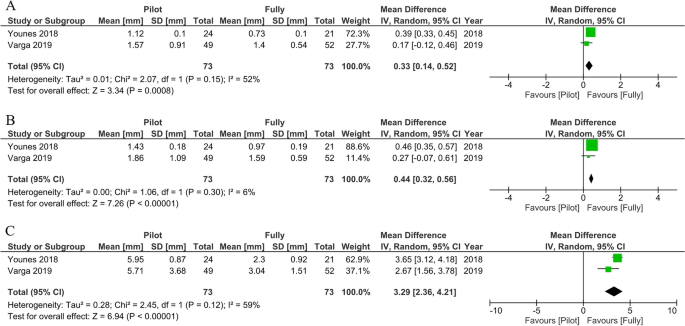
Forest plots of global coronal ( A ), global apical ( B ), and angular deviation ( C ) comparing clinical study of the static system between pilot and fully protocols
On the other hand, one RCT [ 77 ] and one retrospective study [ 60 ] ( n = 177 implants) found no statistically significant difference between the partial and full protocols in global coronal deviation (MD:0.42 mm; 95% CI: -0.47 to 1.30; P = 0.39; I 2 = 95%), global apical deviation (MD:0.47 mm; 95% CI: -0.48 to 1.42; P = 0.34; I 2 = 92%), and angular deviation. (MD:2.17 mm; 95% CI: 0.24 to 4.09; P = 0.03; I 2 = 79%). A high degree of heterogeneity between the studies was found for angular, coronal, and apical deviations (Fig. 14 ).
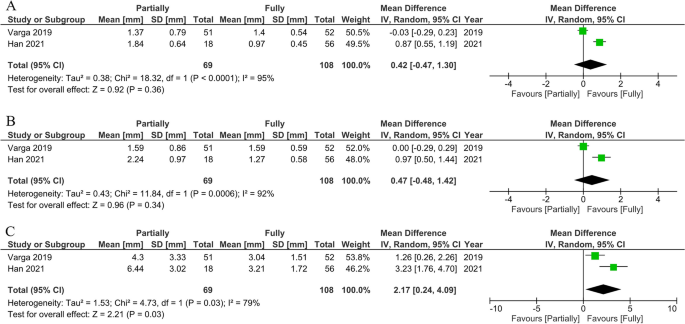
Forest plots of global coronal ( A ), global apical ( B ), and angular deviation ( C ) comparing clinical study of static system between partial and fully protocols
This systematic review evaluated the clinical accuracy of computer-aided static, dynamic, and robot-assisted surgery in implant surgery, along with the related factors. The result showed sixty-eight clinical studies analyzing the accuracy of computerized-implant surgery and determined that the average global coronal deviation, global apical deviation, and angular deviation were 1.11 mm, 1.40 mm, and 3.51˚, respectively. In comparison to the word by Jorba-Garcia et al. [ 10 ], these findings demonstrated a comparable discrepancy in the overall coronal and apical parts, albeit with a slightly lesser angular deviation. This may be due to the wider scope of our systematic review, which included articles specifically focused on robotic CAIS.
Our included studies showed two clinical reports [ 11 , 13 ] assessing the accuracy of robot-assisted implant systems. These reports indicated that this novelty outperformed static and dynamic systems in terms of accuracy and precision. To date, an autonomous robotic system has achieved better 3D accuracy than the Yomi system. However, it should be noted that an autonomous robotic system performing under a maxillary tooth-supported guide may provide a more stable robotic splint base. On the other hand, the Yomi system used both maxillary and mandibular arches, with mucosa-supported guides. Generally, the robot-assisted system still presents some drawbacks, including the inability to operate on patients with limited mouth opening, and cannot be performed by inexperienced surgeons. Due to the lack of comparative cohort studies, it is crucial to take into account that the available data on this technology must be further explored with additional clinical proof.
Regarding the patients’ clinical conditions, our meta-analysis showed no statistically significant differences in coronal, apical, and angular deviation when comparing the maxillary and mandibular arches in dynamic systems. Two studies reported no difference in accuracy when inserting the implant between the upper and lower jaw [ 25 , 68 ]. Nevertheless, there was a slight difference favoring the mandibular osteotomy. In contrast, a meta-analysis reported statistically significant differences in angular deviation favoring the mandibular arch in the case of static systems [ 20 ], explaining that the mandibular architecture is straighter than the maxillary arch, causing difficulties in controlling the angle of the dental bur. Another reason might be that the dense bone in the mandible could aid in restricting guided drilling and implant insertion.
Moreover, a higher bone density facilitates the segmentation step of the CBCT dataset and the registration procedure due to the high contrast of the images [ 60 ]. Stefanelli and colleagues noted that dynamics surgery easily provides direct access to the mandibular and maxillary operation sites. Meanwhile, static surgery performed with a thermoplastic stent [ 25 ] might alleviate the potential error in the maxilla and support our report that there is no difference between the maxilla and mandible. However, the available data in our review are relatively scarce, with few patients. Consequently, more data are needed for further evaluation and research.
Dynamic navigation can improve on computer-guided systems by adjusting the preoperative plan or evaluating the indiscernible vital structures underneath bone in real-time. Therefore, clinicians can perform the flapless protocol regardless of the limited visualization of the implant site. According to Zhou and colleagues [ 20 ], who compared the accuracy of static guided surgery between open-flap or flapless operations, the results showed a significant difference favoring the flapless procedure in terms of the accuracy of implant placement. This result is explained by the instability of a surgical guide when the flap is expanded. In addition to its superior accuracy, flapless surgery may reduce postoperative morbidities such as bleeding and patient discomfort, as well as time consumption [ 89 ]. Our meta-analysis compared open-flap and flapless surgery in the case of dynamic navigation. Dissimilar to the summarized review for guided surgery, there was no significant difference in angular, coronal, and apical deviation between the two methods. Two prospective studies [ 16 , 68 ] showed no significant differences between the groups with no surgical template in the operation area, and the reflected tissue may not be interfered with by the guide. Although flapless surgery is more favorable than open-flap surgery, the operator should cautiously consider the preoperative procedure before launch. In case of an insufficient keratinized mucosa (less than 2 mm), a complete lack of keratinized tissue occurred at the buccal aspect after prosthetic restoration. Therefore, flapless surgery must only be performed if sufficient keratinized tissue is available [ 21 ]. Due to few patients being included in our meta-analysis ( n = 38), further studies must be conducted to allow a conclusive summary.
Different guided surgical protocols were analyzed in our study. The implant protocols are typically categorized into three main types: pilot, partial or half, and complete (full) protocols. The fully-guided protocol, also known as the complete protocol, has been evaluated for its accuracy in various clinical studies. This protocol aids the operator at each surgical step, from the initial osteotomy to the insertion of the implant. Meanwhile, a guided template is only used in the osteotomy, but the operator still installs an implant free handed, which is classified as a partial (half) protocol. A pilot protocol is then used when a clinician wants to initially locate an implant position before drilling and inserting via mental navigation. This meta-analysis review analyzed the accuracy of implant placement using static guided surgery by comparing the three protocols. Our study found significant differences favoring the fully guided protocol in all directions. Other meta-analyses also illustrated the greater accuracy of the full protocol [ 89 , 90 ]. Younes and coworkers explained that significant deviations from virtual planning might occur in every drill sequence from the pilot drill, so the clinician should passively insert the implant to escape these cumulative errors. Another critical procedure that reduces depth deviation uses the stereolithographic template for fully guided surgery [ 76 ]. Interestingly, Varga et al. found statistically significant differences only in terms of angular deviation. Accurate angulation is most important in the case of screw-retained restoration or an angulated abutment because misangulation can detrimentally influence the type of prosthetic restoration [ 77 ].
On the contrary, our meta-analysis revealed no significant difference between partially and fully guided surgery. The clinical studies described a greater accuracy provided by the fully guided protocol than by the partial protocol.Varga and coworkers suggested that “the higher the level of guidance is, the higher the correspondence between the planned and the actual implant position”. The higher correspondence can imply that the fully and partially guided surgery shows a safer guided option in terms of accuracy than pilot-guided or freehand surgery [ 77 ]. On the other hand, a previous meta-analysis [ 20 ] showed a statistically significant greater deviation in all parameters when comparing partially and fully guided protocols. They authors described how insertion by hand instead of a guide led to a more significant error in partially guided surgery. Notably, Zhou and colleagues included former publications that were not the same as those in our review. Therefore, the different results may be caused by the technological improvements in surgical-guided system. Due to the cost-effectiveness of guided surgery and some limitations to this approach, partially guided surgery is acceptable and widely used in clinical applications [ 76 ].
Aside from statics navigations, dynamic navigation can be divided into two protocols: drilling holes and fully guided implant placement. The fully guided protocol uses a dynamic procedure to initially drill the first drill until complete implant installation. Partial guidance refers to an operator performing osteotomy using the dynamic system and the implant seating of at least half of its length by hand [ 15 ]. A previous meta-analysis [ 9 ] reported no statistically significant differences between the partially and fully guided protocols, finding a slight difference favoring fully guided implantation because the fully guided placement proceeded at a slower speed than the drill-hole method. However, further studies should explore the clinical relevance of this comparison.
The 4th EAO consensus conference 2015 [ 91 ] stated the standardized postoperative follow-up of implant-related parameters: the 3D implant position, the peri-implant bone structures and morphology, the mucosa color, contour, and color of the reconstruction, and the extent of the restorative misfit. Moreover, the latest EAO [ 92 ] recommends using patient-reported outcome measurements (PROMs) in all clinical research. PROMS included the oral health impact profile (OHIP), the standardized use of the visual analog scale (VAS) for pain and discomfort, cost–benefit analysis, the time efficiency factor, and complication rates. Nevertheless, most clinical research has been driven by the implant-reported outcomes, especially in accuracy, without focusing on PROMs, which should constitute the virtual endpoint of clinical trials.
CBCT is usually an effective tool for assessing the accuracy measurement in clinical research for evaluating hard tissue, soft tissue, and implant position in 3D features. Despite the advantages of CBCT over other measurements, the ALARA (as low as reasonably achievable) rule should be followed for patient safety [ 91 ]. Currently, the analysis of implant placement position can be carried out with the utilization of a model scanner, intra-oral scanner, and CBCT. Akira and colleagues [ 23 ] investigated the consistency of measurements and the degree of shrinkage across three different modalities. The findings indicated that the shrinkage in CBCT was the most significant among the three modalities, primarily due to factors inherent to the system. They also stated that the data matching between CBCT and scanner measurements necessitates careful consideration regarding the accuracy of the values obtained with these devices. Furthermore, Derksen and coworkers reported that postoperative intraoral scan and postoperative CBCT scan techniques had similar accuracy outcomes. They recommended that more studies be conducted to confirm this hypothesis [ 21 ].
The limitation of this systematic review and meta-analysis involves the heterogeneity among the included studies’ static, dynamic, and robotic systems, regardless of the different study designs. The potential factors in each system are the lists explained earlier that contribute to the cumulative errors. Apart from these, possible factors include guide support, guide stability, the restriction of access during surgery (location and limited mouth opening), the movement of the patient, the edentulous space, the time of placement, the characteristics of the implant (material, diameter, length), the operator’s experience, or different postoperative assessment, which may result in an undesirable outcome. Moreover, the quality of studies, including considering a prospective study and a case series, should be realized. Overall, the randomized controlled trials appeared to be of some concern, whereas non-randomized controlled trials were classified as high quality. A common consensus should be followed to avoid potential bias in applying this study’s results.
Conclusions
Within the limitations of our reviews, the robotic system consistently exhibited the least amount of deviation, followed by the dynamic and static systems. Nevertheless, the increased accuracy achieved via robotic guided surgery should be taken with caution until further research and technology is available. Considering the relevant factors, no significant differences were found between the arch and flap approaches in the dynamic systems. In the case of the static systems, there were statistically significant differences observed between the pilot and fully guided protocols, but no significant differences were found between the partially and fully guided protocols. Moreover, it is the consensus of several studies that a fully guided protocol is the gold standard in clinical practice. Future clinical research should focus on exploring the application of robot-guided systems in clinical settings to enhance the accuracy of implant placement and PROMs utilizing implant-assisted systems.
Available of data and materials
All data generated and analyzed for the review are available upon request from the authors (Corresponding author: [email protected] ).
Abbreviations
Cone-beam computed tomography (CBCT)
Static computer-aided implant surgery
Dynamic computer-aided implant surgery
Digital imaging and communications in medicine
Standard Template Library
Yang Y, Hu H, Zeng M, et al. The survival rates and risk factors of implants in the early stage: a retrospective study. BMC Oral Health. 2021;21:293.
Article PubMed PubMed Central Google Scholar
Busenlechner D, Fürhauser R, Haas R, Watzek G, Mailath G, Pommer B. Long-term implant success at the Academy for Oral Implantology: 8-year follow-up and risk factor analysis. J Periodontal Implant Sci. 2014;44(3):102–8.
Garber DA, Belser UC. Restoration-driven implant placement with restoration-generated site development. Compend Contin Educ Dent. 1995;16(8):798–802.
Google Scholar
Belser UC, Bernard JP, Buser D. Implant-supported restorations in the anterior region: prosthetic considerations. Pract Periodontics Aesthet Dent. 1996;8(9):875–83.
CAS PubMed Google Scholar
Putra RH, Cooray U, Nurrachman AS, Yoda N, Judge R, Putri DK, Astuti ER. Radiographic alveolar bone assessment in correlation with primary implant stability: A systematic review and meta-analysis. Clin Oral Implants Res. 2024;35(1):1–20.
Article PubMed Google Scholar
Tahmaseb A, Wismeijer D, Coucke W, Derksen W. Computer technology applications in surgical implant dentistry: a systematic review. Int J Oral Maxillofac Implants. 2014;29(Suppl):25–42.
Zhang S, Cai Q, Chen W, Lin Y, Gao Y, Wu D, Chen J. Accuracy of implant placement via dynamic navigation and autonomous robotic computer-assisted implant surgery methods: A retrospective study. Clin Oral Implants Res. 2024;35(2):220–9.
Cassetta M, Altieri F, Giansanti M, Bellardini M, Brandetti G, Piccoli L. Is there a learning curve in static computer-assisted implant surgery? A prospective clinical study. Int J Oral Maxillofac Surg. 2020;49(10):1335–42.
Article CAS PubMed Google Scholar
Wei SM, Zhu Y, Wei JX, Zhang CN, Shi JY, Lai HC. Accuracy of dynamic navigation in implant surgery: A systematic review and meta-analysis. Clin Oral Implants Res. 2021;32(4):383–93.
Jorba-García A, González-Barnadas A, Camps-Font O, Figueiredo R, Valmaseda-Castellón E. Accuracy assessment of dynamic computer-aided implant placement: a systematic review and meta-analysis. Clin Oral Investig. 2021;25(5):2479–94.
Bolding SL, Reebye UN. Accuracy of haptic robotic guidance of dental implant surgery for completely edentulous arches. J Prosthet Dent. 2022;128(4):639–47.
Yimarj P, Subbalekha K, Dhanesuan K, Siriwatana K, Mattheos N, Pimkhaokham A. Comparison of the accuracy of implant position for two-implants supported fixed dental prosthesis using static and dynamic computer-assisted implant surgery: A randomized controlled clinical trial. Clin Implant Dent Relat Res. 2020;22(6):672–8.
Yang S, Chen J, Li A, Li P, Xu S. Autonomous Robotic Surgery for Immediately Loaded Implant-Supported Maxillary Full-Arch Prosthesis: A Case Report. J Clin Med. 2022;11(21):6594.
Sigcho López DA, García I, Da Silva SG, Cruz LD. Potential Deviation Factors Affecting Stereolithographic Surgical Guides: A Systematic Review. Implant Dent. 2019;28(1):68–73.
Block MS, Emery RW, Cullum DR, Sheikh A. Implant Placement Is More Accurate Using Dynamic Navigation. J Oral Maxillofac Surg. 2017;75(7):1377–86.
Pellegrino G, Taraschi V, Andrea Z, Ferri A, Marchetti C. Dynamic navigation: a prospective clinical trial to evaluate the accuracy of implant placement. Int J Comput Dent. 2019;22(2):139–47.
PubMed Google Scholar
Chen W, Al-Taezi KA, Chu CH, Shen Y, Wu J, Cai K, Chen P, Tang C. Accuracy of dental implant placement with a robotic system in partially edentulous patients: A prospective, single-arm clinical trial. Clin Oral Implants Res. 2023;34(7):707–18.
D’Haese J, Van De Velde T, Elaut L, De Bruyn H. A prospective study on the accuracy of mucosally supported stereolithographic surgical guides in fully edentulous maxillae. Clin Implant Dent Relat Res. 2012;14(2):293–303.
Pettersson A, Komiyama A, Hultin M, Nasstrom K, Klinge B. Accuracy of virtually planned and template guided implant surgery on edentate patients. Clin Implant Dent Relat Res. 2012;14(4):527–37.
Zhou W, Liu Z, Song L, Kuo CL, Shafer DM. Clinical Factors Affecting the Accuracy of Guided Implant Surgery-A Systematic Review and Meta-analysis. J Evid Based Dent Pract. 2018;18(1):28–40.
Derksen W, Wismeijer D, Flugge T, Hassan B, Tahmaseb A. The accuracy of computer-guided implant surgery with tooth-supported, digitally designed drill guides based on CBCT and intraoral scanning. A prospective cohort study. Clin Oral Implants Res. 2019;30(10):1005–15.
Kiatkroekkrai P, Takolpuckdee C, Subbalekha K, Mattheos N, Pimkhaokham A. Accuracy of implant position when placed using static computer-assisted implant surgical guides manufactured with two different optical scanning techniques: a randomized clinical trial. Int J Oral Maxillofac Surg. 2020;49(3):377–83.
Komuro A, Yamada Y, Uesugi S, Terashima H, Kimura M, Kishimoto H, Iida T, Sakamoto K, Okuda K, Kusano K, Baba S, Sakamoto T. Accuracy and dimensional reproducibility by model scanning, intraoral scanning, and CBCT imaging for digital implant dentistry. Int J Implant Dent. 2021;7(1):63.
Chackartchi T, Romanos GE, Parkanyi L, Schwarz F, Sculean A. Reducing errors in guided implant surgery to optimize treatment outcomes. Periodontol 2000. 2022;88(1):64–72.
Stefanelli LV, Mandelaris GA, DeGroot BS, Gambarini G, De Angelis F, Di Carlo S. Accuracy of a Novel Trace-Registration Method for Dynamic Navigation Surgery. Int J Periodontics Restorative Dent. 2020;40(3):427–35.
Aydemir CA, Arisan V. Accuracy of dental implant placement via dynamic navigation or the freehand method: A split-mouth randomized controlled clinical trial. Clin Oral Implants Res. 2020;31(3):255–63.
Tao B, Feng Y, Fan X, Zhuang M, Chen X, Wang F, Wu Y. Accuracy of dental implant surgery using dynamic navigation and robotic systems: An in vitro study. J Dent. 2022;123:104170.
Yan B, Zhang W, Cai L, et al. Optics-guided Robotic System for Dental Implant Surgery. Chin J Mech Eng. 2022;35:55.
Article Google Scholar
Tahmaseb A, Wu V, Wismeijer D, Coucke W, Evans C. The accuracy of static computer-aided implant surgery: A systematic review and meta-analysis. Clin Oral Implants Res. 2018;29(16):416–35.
Van Assche N, van Steenberghe D, Quirynen M, Jacobs R. Accuracy assessment of computer-assisted flapless implant placement in partial edentulism. J Clin Periodontol. 2010;37(4):398–403.
Arisan V, Karabuda ZC, Ozdemir T. Accuracy of two stereolithographic guide systems for computer-aided implant placement: a computed tomography-based clinical comparative study. J Periodontol. 2010;81(1):43–51.
Cassetta M, Stefanelli LV, Giansanti M, Di Mambro A, Calasso S. Depth deviation and occurrence of early surgical complications or unexpected events using a single stereolithographic surgi-guide. Int J Oral Maxillofac Surg. 2011;40(12):1377–87.
Cassetta M, Stefanelli LV, Giansanti M, Calasso S. Accuracy of implant placement with a stereolithographic surgical template. Int J Oral Maxillofac Implants. 2012;27(3):655–63.
Cassetta M, Pompa G, Di Carlo S, Piccoli L, Pacifici A, Pacifici L. The influence of smoking and surgical technique on the accuracy of mucosa-supported stereolithographic surgical guide in complete edentulous upper jaws. Eur Rev Med Pharmacol Sci. 2012;16(11):1546–53.
Cassetta M, Stefanelli LV, Giansanti M, Di Mambro A, Calasso S. Accuracy of a computer-aided implant surgical technique. Int J Periodontics Restorative Dent. 2013;33(3):317–25.
Cassetta M, Giansanti M, Di Mambro A, Calasso S, Barbato E. Accuracy of two stereolithographic surgical templates: a retrospective study. Clin Implant Dent Relat Res. 2013;15(3):448–59.
Cassetta M, Di Mambro A, Giansanti M, Stefanelli LV, Cavallini C. The intrinsic error of a stereolithographic surgical template in implant guided surgery. Int J Oral Maxillofac Surg. 2013;42(2):264–75.
Van de Wiele G, Teughels W, Vercruyssen M, Coucke W, Temmerman A, Quirynen M. The accuracy of guided surgery via mucosa-supported stereolithographic surgical templates in the hands of surgeons with little experience. Clin Oral Implants Res. 2015;26(12):1489–94.
Testori T, Robiony M, Parenti A, Luongo G, Rosenfeld AL, Ganz SD, et al. Evaluation of accuracy and precision of a new guided surgery system: a multicenter clinical study. Int J Periodontics Restorative Dent. 2014;34(3):s59-69.
Verhamme LM, Meijer GJ, Berge SJ, Soehardi RA, Xi T, de Haan AF, et al. An Accuracy Study of Computer-Planned Implant Placement in the Augmented Maxilla Using Mucosa-Supported Surgical Templates. Clin Implant Dent Relat Res. 2015;17(6):1154–63.
Alzoubi F, Massoomi N, Nattestad A. Accuracy Assessment of Immediate and Delayed Implant Placements Using CAD/CAM Surgical Guides. J Oral Implantol. 2016;42(5):391–8.
Cassetta M, Bellardini M. How much does experience in guided implant surgery play a role in accuracy? A randomized controlled pilot study. Int J Oral Maxillofac Surg. 2017;46(7):922–30.
Horwitz J, Machtei EE, Zigdon-Giladi H. Clinical accuracy of a novel open-lattice-frame implant positioning system: a case series. Quintessence Int. 2017;48:33–9.
Albiero AM, Benato R, Benato A, Degidi M. Use of intraoral welding to increase the predictability of immediately loaded computer-guided implants. Int J Periodontics Restorative Dent. 2017;37(4):591–8.
Schnutenhaus S, Edelmann C, Rudolph H, Dreyhaupt J, Luthardt RG. 3D accuracy of implant positions in template-guided implant placement as a function of the remaining teeth and the surgical procedure: a retrospective study. Clin Oral Investig. 2018;22(6):2363–72.
Schnutenhaus S, von Koenigsmarck V, Blender S, Ambrosius L, Luthardt RG, Rudolph H. Precision of sleeveless 3D drill guides for insertion of one-piece ceramic implants: a prospective clinical trial. Int J Comput Dent. 2018;21(2):97–105.
Albiero AM, Quartuccio L, Benato A, Benato R. Accuracy of Computer-Guided Flapless Implant Surgery in Fully Edentulous Arches and in Edentulous Arches With Fresh Extraction Sockets. Implant Dent. 2019;28(3):256–64.
Schelbert T, Gander T, Blumer M, Jung R, Rucker M, Rostetter C. Accuracy of Computer-Guided Template-Based Implant Surgery: A Computed Tomography-Based Clinical Follow-Up Study. Implant Dent. 2019;28(6):556–63.
Zhou M, Zhou H, Li SY, Yang XB, Geng YM, Che YJ. Accuracy of Implant Placement Guided with Surgical Template: An In Vitro and In Vivo Study. Int J Periodontics Restorative Dent. 2021;41(2):e55–62.
Skjerven H, Riis UH, Herlofsson BB, Ellingsen JE. In Vivo Accuracy of Implant Placement Using a Full Digital Planning Modality and Stereolithographic Guides. Int J Oral Maxillofac Implants. 2019;34(1):124–32.
Monaco C, Arena A, Corsaletti L, Santomauro V, Venezia P, Cavalcanti R, et al. 2D/3D accuracies of implant position after guided surgery using different surgical protocols: A retrospective study. J Prosthodont Res. 2020;64(4):424–30.
Suksod N, Kunavisarut C, Kitisubkanchana J. Accuracy of computer-guided implantation in the placement of one-piece ceramic dental implants in the anterior region: A prospective clinical study. PLoS One. 2020;15(9):e0237229.
Article CAS PubMed PubMed Central Google Scholar
Chai J, Liu X, Schweyen R, Setz J, Pan S, Liu J, et al. Accuracy of implant surgical guides fabricated using computer numerical control milling for edentulous jaws: a pilot clinical trial. BMC Oral Health. 2020;20(1):288.
Kivovics M, Penzes D, Nemeth O, Mijiritsky E. The Influence of Surgical Experience and Bone Density on the Accuracy of Static Computer-Assisted Implant Surgery in Edentulous Jaws Using a Mucosa-Supported Surgical Template with a Half-Guided Implant Placement Protocol-A Randomized Clinical Study. Materials (Basel). 2020;13(24):5759.
Article ADS CAS PubMed Google Scholar
Zhang X, Wang M, Mo A. An alternative method for immediate implant-supported restoration of anterior teeth assisted by fully guided templates: A clinical study. J Prosthet Dent. 2021;126(5):636–45.
Cunha RM, Souza FA, Hadad H, Poli PP, Maiorana C, Carvalho PSP. Accuracy evaluation of computer-guided implant surgery associated with prototyped surgical guides. J Prosthet Dent. 2021;125(2):266–72.
Cho JY, Kim SB, Ryu J. The accuracy of a partially guided system using an in-office 3D-printed surgical guide for implant placement. Int J Comput Dent. 2021;24(1):19–27.
Gargallo-Albiol J, Zilleruelo-Pozo MJ, Lucas-Taulé E, Muñoz-Peñalver J, Paternostro-Betancourt D, Hernandez-Alfaro F. Accuracy of static fully guided implant placement in the posterior area of partially edentulous jaws: a cohort prospective study. Clin Oral Investig. 2022;26(3):2783–91.
Cristache CM, Burlibasa M, Tudor I, Totu EE, Di Francesco F, Moraru L. Accuracy, Labor-Time and Patient-Reported Outcomes with Partially versus Fully Digital Workflow for Flapless Guided Dental Implants Insertion-A Randomized Clinical Trial with One-Year Follow-Up. J Clin Med. 2021;10(5):1102.
Han YT, Lin WC, Fan FY, Chen CL, Lin CC, Cheng HC. Comparison of Dental Surface Image Registration and Fiducial Marker Registration: An In Vivo Accuracy Study of Static Computer-Assisted Implant Surgery. J Clin Med. 2021;10(18):4183.
Schnutenhaus S, Edelmann C, Rudolph H. Does the macro design of an implant affect the accuracy of template-guided implantation? A prospective clinical study. Int J Implant Dent. 2021;7(1):42.
Matsumura A, Nakano T, Ono S, Kaminaka A, Yatani H, Kabata D. Multivariate analysis of causal factors influencing accuracy of guided implant surgery for partial edentulism: a retrospective clinical study. Int J Implant Dent. 2021;7(1):28.
De Souza AB, Kang M, Negreiros WM, El-Rafie K, Finkelman M, Papaspyridakos P. A comparative retrospective study of different surgical guide designs for static computer-assisted implant surgery in posterior single edentulous sites. Clin Oral Implant Res. 2022;33(1):45–52.
Sun Y, Ding Q, Yuan F, Zhang L, Sun Y, Xie Q. Accuracy of a chairside, fused deposition modeling three-dimensional-printed, single tooth surgical guide for implant placement: A randomized controlled clinical trial. Clin Oral Implant Res. 2022;33(10):1000–9.
Article CAS Google Scholar
Orban K, Varga E Jr, Windisch P, Braunitzer G, Molnar B. Accuracy of half-guided implant placement with machine-driven or manual insertion: A prospective, randomized clinical study. Clin Oral Invest. 2022;26(1):1035–43.
Ngamprasertkit C, Aunmeungthong W, Khongkhunthian P. The implant position accuracy between using only surgical drill guide and surgical drill guide with implant guide in fully digital workflow: a randomized clinical trial. Oral Maxillofac Surg. 2022;26(2):229–37.
Zhu F, Mao M, Zhu H, Chen Y, You J, Pan H. Comparison of Positioning Accuracy Between 2 Different Implant Systems Using Mucosa-Supported Surgical Templates: A Retrospective Clinical Study. Journal of Oral Implantology. 2022;48(1):15–20.
Edelmann C, Wetzel M, Knipper A, Luthardt RG, Schnutenhaus S. Accuracy of Computer-Assisted Dynamic Navigation in Implant Placement with a Fully Digital Approach: A Prospective Clinical Trial. J Clin Med. 2021;10(9):1808.
Wei SM, Shi JY, Qiao SC, Zhang X, Lai HC, Zhang XM. Accuracy and primary stability of tapered or straight implants placed into fresh extraction socket using dynamic navigation: a randomized controlled clinical trial. Clin Oral Investig. 2022;26(3):2733–41.
Ma F, Sun F, Wei T, Ma Y. Comparison of the accuracy of two different dynamic navigation system registration methods for dental implant placement: A retrospective study. Clin Implant Dent Relat Res. 2022;24(3):352–60.
van Hooft J, et al. Intraoral Scanning as an Alternative to Evaluate the Accuracy of Dental Implant Placements in Partially Edentate Situations: A Prospective Clinical Case Series. J Clin Med. 2022;11(19):5876.
Vercruyssen M, Cox C, Coucke W, Naert I, Jacobs R, Quirynen M. A randomized clinical trial comparing guided implant surgery (bone- or mucosa-supported) with mental navigation or the use of a pilot-drill template. J Clin Periodontol. 2014;41(7):717–23.
Shen P, Zhao J, Fan L, Qiu H, Xu W, Wang Y, et al. Accuracy evaluation of computer-designed surgical guide template in oral implantology. J Craniomaxillofac Surg. 2015;43(10):2189–94.
Smitkarn P, Subbalekha K, Mattheos N, Pimkhaokham A. The accuracy of single-tooth implants placed using fully digital-guided surgery and freehand implant surgery. J Clin Periodontol. 2019;46(9):949–57.
Schneider D, Sancho-Puchades M, Mir-Mari J, Muhlemann S, Jung R, Hammerle C. A Randomized Controlled Clinical Trial Comparing Conventional and Computer-Assisted Implant Planning and Placement in Partially Edentulous Patients. Part 4: Accuracy of Implant Placement. Int J Periodontics Restorative Dent. 2019;39(4):e111–22.
Younes F, Cosyn J, De Bruyckere T, Cleymaet R, Bouckaert E, Eghbali A. A randomized controlled study on the accuracy of free-handed, pilot-drill guided and fully guided implant surgery in partially edentulous patients. J Clin Periodontol. 2018;45(6):721–32.
Varga E Jr, Antal M, Major L, Kiscsatari R, Braunitzer G, Piffko J. Guidance means accuracy: A randomized clinical trial on freehand versus guided dental implantation. Clin Oral Implants Res. 2020;31(5):417–30.
Tencati E, Moy P. Accuracy of Dynamic Computer-Aided Navigation System for Dental Implant Placement. J Oral Maxillofac Surg. 2019;77:e68–9.
Kaewsiri D, Panmekiate S, Subbalekha K, Mattheos N, Pimkhaokham A. The accuracy of static vs. dynamic computer-assisted implant surgery in single tooth space: A randomized controlled trial. Clin Oral Implants Res. 2019;30(6):505–14.
Nomiyama LM, Matumoto EK, Corrêa MG, Cirano FR, Ribeiro FV, Pimentel SP, Casati MZ. Comparison between flapless-guided and conventional surgery for implant placement: a 12-month randomized clinical trial. Clin Oral Investig. 2022;27(4):1665–79.
Wei SM, Li Y, Deng K, Lai HC, Tonetti MS, Shi JY. Does machine-vision-assisted dynamic navigation improve the accuracy of digitally planned prosthetically guided immediate implant placement? A randomized controlled trial. Clin Oral Implant Res. 2022;33(8):804–15.
Feng Y, Su Z, Mo A, Yang X. Comparison of the accuracy of immediate implant placement using static and dynamic computer-assisted implant system in the esthetic zone of the maxilla: a prospective study. International Journal of Implant Dentistry. 2022;8(1):65.
Jaemsuwan S, Arunjaroensuk S, Kaboosaya B, Subbalekha K, Mattheos N, Pimkhaokham A. Comparison of the accuracy of implant position among freehand implant placement, static and dynamic computer-assisted implant surgery in fully edentulous patients: a non-randomized prospective study. Int J Oral Maxillofac Surg. 2022;52(2):264–71.
Sterne JA, Savović J, Page MJ, Elbers RG, Blencowe NS, Boutron I, et al. RoB 2: a revised tool for assessing risk of bias in randomised trials. BMJ. 2019;366:l4898.
Sterne JA, Hernán M A, Reeves B C, Savović J, Berkman N D, Viswanathan M, et al. ROBINS-I: a tool for assessing risk of bias in non-randomised studies of interventions. BMJ. 2016;355:i4919.
Moola S, Munn Z, Tufanaru C, Aromataris E, Sears K, Sfetcu R, et al. Chapter 7: Systematic reviews of etiology and risk. In: Aromataris E, Munn Z (Editors). JBI Manual for Evidence Synthesis. JBI, 2020. https://synthesismanual.jbi.globa .
Suurmond R, van Rhee H, Hak T. Introduction, comparison, and validation of Meta-Essentials: a free and simple tool for meta-analysis. Research synthesis methods. 2017;8(4):537–53.
Higgins JPT, Thomas J, Chandler J, Cumpston M, Li T, Page MJ et al. Cochrane handbook for systematic reviews of interventions. Wiley, 2019. p. 694.
Bover-Ramos F, Viña-Almunia J, Cervera-Ballester J, Peñarrocha-Diago M, García-Mira B. Accuracy of Implant Placement with Computer-Guided Surgery: A Systematic Review and Meta-Analysis Comparing Cadaver, Clinical, and In Vitro Studies. Int J Oral Maxillofac Implants. 2018;33(1):101–15.
Putra RH, Yoda N, Astuti ER, Sasaki K. The accuracy of implant placement with computer-guided surgery in partially edentulous patients and possible influencing factors: A systematic review and meta-analysis. J Prosthodont Res. 2022;66(1):29–39.
Hämmerle CH, Cordaro L, van Assche N, Benic GI, Bornstein M, Gamper F, et al. Digital technologies to support planning, treatment, and fabrication processes and outcome assessments in implant dentistry. Summary and consensus statements. The 4th EAO consensus conference 2015. Clin Oral Implants Res. 2015;26(11):97–101.
Wismeijer D, et al. Group 5 ITI Consensus Report: Digital technologies. Clin Oral Implants Res. 2018;29(16):436–42.
Download references
Acknowledgements
The researchers would like to thank Dr. Watcharapong Tonprasong (Department of Restorative Dentistry, Faculty of Dentistry, Naresuan University, Phitsanulok, Thailand) for supporting and suggesting quality assessment tools.
This research was supported by the TA/RA Scholarship from Graduate School, Chiang Mai University.
This study is partially funded by Chiang Mai University, and Faculty of Dentistry, Chiang Mai University, Thailand.
Author information
Authors and affiliations.
Department of Prosthodontics, Faculty of Dentistry, Chiang Mai University, Chiang Mai, 50200, Thailand
Angkoon Khaohoen, Pisaisit Chaijareenont & Pimduen Rungsiyakull
Department of Oral and Maxillofacial Surgery, Faculty of Dentistry, Chiang Mai University, Chiang Mai, 50200, Thailand
Warit Powcharoen
Department of Restorative Dentistry, Faculty of Dentistry, Naresuan University, Phitsanulok, 65000, Thailand
Tanapon Sornsuwan
Department of Mechanical Engineering, Faculty of Engineering, Chiang Mai University, Chiang Mai, 50200, Thailand
Chaiy Rungsiyakull
You can also search for this author in PubMed Google Scholar
Contributions
Conceptualization, A.K. and P.R.; methodology, A.K.,W.P., and P.R.; software, A.K. and W.P.; validation, P.R., A.K. and C.R.; formal analysis, A.K., P.R. and W.P.; investigation, A.K., P.R.; resources, A.K., P.R. and C.R.; data curation, A.K., P.R.; writing—original draft preparation, A.K.; writing—review and editing, A.K., P.R., W.P., P.C., T.S.; visualization, P.R., C.R., T.S.; supervision, P.R.; project administration, P.R.; funding acquisition, P.R. All authors have read and agreed to the published version of the manuscript.
Corresponding author
Correspondence to Pimduen Rungsiyakull .
Ethics declarations
Ethics approval and consent to participate.
Not applicable.
Consent for publication
Competing interests.
The authors declare no competing interests.
Additional information
Publisher’s note.
Springer Nature remains neutral with regard to jurisdictional claims in published maps and institutional affiliations.
Rights and permissions
Open Access This article is licensed under a Creative Commons Attribution 4.0 International License, which permits use, sharing, adaptation, distribution and reproduction in any medium or format, as long as you give appropriate credit to the original author(s) and the source, provide a link to the Creative Commons licence, and indicate if changes were made. The images or other third party material in this article are included in the article's Creative Commons licence, unless indicated otherwise in a credit line to the material. If material is not included in the article's Creative Commons licence and your intended use is not permitted by statutory regulation or exceeds the permitted use, you will need to obtain permission directly from the copyright holder. To view a copy of this licence, visit http://creativecommons.org/licenses/by/4.0/ . The Creative Commons Public Domain Dedication waiver ( http://creativecommons.org/publicdomain/zero/1.0/ ) applies to the data made available in this article, unless otherwise stated in a credit line to the data.
Reprints and permissions
About this article
Cite this article.
Khaohoen, A., Powcharoen, W., Sornsuwan, T. et al. Accuracy of implant placement with computer-aided static, dynamic, and robot-assisted surgery: a systematic review and meta-analysis of clinical trials. BMC Oral Health 24 , 359 (2024). https://doi.org/10.1186/s12903-024-04033-y
Download citation
Received : 09 October 2023
Accepted : 14 February 2024
Published : 21 March 2024
DOI : https://doi.org/10.1186/s12903-024-04033-y
Share this article
Anyone you share the following link with will be able to read this content:
Sorry, a shareable link is not currently available for this article.
Provided by the Springer Nature SharedIt content-sharing initiative
- Robotic guide surgery
- Image-guided surgery
- Computer-assisted implantation
- Surgical navigation
- Dental implants
- Systematic review
BMC Oral Health
ISSN: 1472-6831
- Submission enquiries: [email protected]
- General enquiries: [email protected]
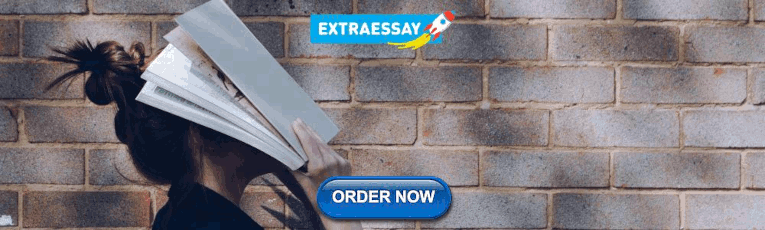
IMAGES
VIDEO
COMMENTS
A mixed method combining systematic mapping and systematic review were used in the study. Ninety-three articles published in Social Sciences Citation Index (SSCI) indexed journals and meeting the specified criteria were analyzed using a systematic mapping process. The results showed that 40 out of 93 articles did not include any learning theory.
Abstract. This study, which systematically examines educational robotics and robots (ERR), has two purposes. (1) Classifying the research on the ERR to identify research trends and gaps, (2 ...
This study, which systematically examines educational robotics and robots (ERR), has two purposes. (1) Classifying the research on the ERR to identify research trends and gaps, (2) Summarizing the experimental findings related to ERR and to interpret them according to the claims in the literature. A mixed method combining systematic mapping and systematic review were used in the study.
ABSTRACT This study, which systematically examines educational robotics and robots (ERR), has two purposes. (1) Classifying the research on the ERR to identify research trends and gaps, (2) Summarizing the experimental findings related to ERR and to interpret them according to the claims in the literature. A mixed method combining systematic ...
1. Introduction. Robots are recognized as an innovative learning tool. Many researchers have indicated that this new technique could change the current educational approaches and facilitate students' learning in different learning environments (Anwar et al., 2019; Evripidou et al., 2020; Lin et al., 2022).Evripidou et al. (2020) pointed out that educational robots can enhance a variety of ...
REVIEW ARTICLE A systematic review study on educational robotics and robots Nilüfer Atman Uslu a, Gulay Öztüre Yavuzb and Yasemin Koçak Usluel b aComputer Education and Instructional Technology Department, Manisa Celal Bayar University, Turkey; bComputer Education and Instructional Technology Department, Hacettepe University, Turkey ABSTRACT This study, which systematically examines ...
Evidence Acquisition: This is a systematic review study conducted in PubMed/Medline, Scopus, Embase, Cochrane Library, Science Direct, and Web of Science (ISI) databases on October 8 2020. Also, we searched Google Scholar to find all in-press articles. To extract data, a checklist was used, which included the author's name, year of ...
A Systematic Review of Studies on Educational Robotics. Journal of Pre-College Engineering Education Research (J-PEER), 9(2), Article 2. This document has been made available through Purdue e-Pubs, a service of the Purdue University Libraries. Please contact [email protected] for additional information.
The literature has evidence of existing studies on robotics at the elementary and secondary levels. The study by (Amo et al., 2021) ... Based on the findings of this systematic review, educational robots are beneficial for the general development of multiple competencies and cognition at an early age and complement a better understanding of ...
This study conducted a systematic and thematic review on existing literature in robotics education using robotics kits (not social robots) for young children (Pre-K and kindergarten through 5th grade). This study investigated: (1) the definition of robotics education; (2) thematic patterns of key findings; and (3) theoretical and methodological traits. The results of the review present a ...
There has been a steady increase in the number of studies investigating educational robotics and its impact on academic and social skills of young learners. Educational robots are used both in and out of school environments to enhance K-12 students' interest, engagement, and academic achievement in various fields of STEM education. Some prior studies show evidence for the general benefits ...
This systematic review evaluated existing studies in improving K-12 students' computational thinking and STEM attitudes. Research articles published between 2010 and 2019 were collated from major databases according to six criteria, and 17 studies were eligible. ... (2016). Learning by robotics: The impact of educational robots on learning ...
Abstract: Abstract Background The application of artificial intelligence (AI) in STEM education (AI-STEM), as an emerging field, is confronted with a challenge of integrating diverse AI techniques and complex educational elements to meet instructional and learning needs. To gain a comprehensive understanding of AI applications in STEM education, this study conducted a systematic review to ...
Numerous studies have been conducted to investigate the potential effect of educational robots, but what appears to be missing is an up-to-date and thorough review of the learning effectiveness of educational robots and the various influencing factors. In this study, a meta-analysis was conducted to systematically synthesize studies' findings on the effects of educational robots on students ...
By providing students with a highly interactive and hands-on learning experience, robotics promises to inspire a new generation of mathematical learning. This paper aims to review the empirical evidence on the application of robotics in mathematics education and to define future research perspectives of robot-assisted mathematics education. After a systematic search in online database via ...
A systematic review of the literature on K-12 educational robotics found 147 studies published from the years 2000 to 2018 and classified these studies under five themes: general effectiveness of educational robotics; students' learning and transfer skills; creativity and motivation; diversity and broadening participation; and teachers' professional development. Abstract There has been a ...
This systematic review analyzes the presence that technology, robotics specifically, has had on our educational system in recent years. Some keywords have been chosen, and screening has been ...
Received 29 June 2021 Accepted 23 December 2021 KEYWORDS Educational robotics; educational robots; systematic mapping; systematic review 1. Introduction Educational robotics and robots (ERR) are recognized as innovative learning tools that can transform education and support students in many learning contexts (Evripidou et al., 2020).
A literature review on educational robotics simulators with Graphical User Interfaces (GUIs) is carried out and seventeen simulators emphasizing key features such as: user's age, robot''s type and programming language, development platform, capabilities, and scope of the simulator are presented.
According to the results of the research, most theses were carried out in 2019 and educational robotics was mostly studied in the master's theses. Experimental design with the quantitative method and the quasi-experimental pre-test post-test control group was widely used as research methods.
There has been a steady increase in the number of studies investigating educational robotics and its impact on academic and social skills of young learners. Educational robots are used both in and out of school environments to enhance K-12 students' interest, engagement, and academic achievement in various fields of STEM education. Some prior studies show evidence for the general benefits of ...
Of the total studies included, 17 (61%) used an educational robot, within that number, 3 studies used built and compact robots, and for this reason they were calculated twice within the subcategory. Only 6 researches (21%) used a social robot, and 5 researches (18%) did not use any robotic devices. TABLE 10. Table 10.
This systematic review explores the accuracy of computerized guided implant placement including computer-aided static, dynamic, and robot-assisted surgery. An electronic search up to February 28, 2023, was conducted using the PubMed, Embase, and Scopus databases using the search terms "surgery", "computer-assisted", "dynamic computer-assisted", "robotic surgical procedures ...