Scope and Delimitations in Research
Delimitations are the boundaries that the researcher sets in a research study, deciding what to include and what to exclude. They help to narrow down the study and make it more manageable and relevant to the research goal.
Updated on October 19, 2022

All scientific research has boundaries, whether or not the authors clearly explain them. Your study's scope and delimitations are the sections where you define the broader parameters and boundaries of your research.
The scope details what your study will explore, such as the target population, extent, or study duration. Delimitations are factors and variables not included in the study.
Scope and delimitations are not methodological shortcomings; they're always under your control. Discussing these is essential because doing so shows that your project is manageable and scientifically sound.
This article covers:
- What's meant by “scope” and “delimitations”
- Why these are integral components of every study
- How and where to actually write about scope and delimitations in your manuscript
- Examples of scope and delimitations from published studies
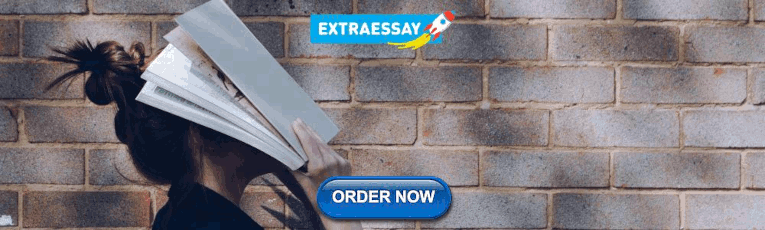
What is the scope in a research paper?
Simply put, the scope is the domain of your research. It describes the extent to which the research question will be explored in your study.
Articulating your study's scope early on helps you make your research question focused and realistic.
It also helps decide what data you need to collect (and, therefore, what data collection tools you need to design). Getting this right is vital for both academic articles and funding applications.
What are delimitations in a research paper?
Delimitations are those factors or aspects of the research area that you'll exclude from your research. The scope and delimitations of the study are intimately linked.
Essentially, delimitations form a more detailed and narrowed-down formulation of the scope in terms of exclusion. The delimitations explain what was (intentionally) not considered within the given piece of research.
Scope and delimitations examples
Use the following examples provided by our expert PhD editors as a reference when coming up with your own scope and delimitations.
Scope example
Your research question is, “What is the impact of bullying on the mental health of adolescents?” This topic, on its own, doesn't say much about what's being investigated.
The scope, for example, could encompass:
- Variables: “bullying” (dependent variable), “mental health” (independent variable), and ways of defining or measuring them
- Bullying type: Both face-to-face and cyberbullying
- Target population: Adolescents aged 12–17
- Geographical coverage: France or only one specific town in France
Delimitations example
Look back at the previous example.
Exploring the adverse effects of bullying on adolescents' mental health is a preliminary delimitation. This one was chosen from among many possible research questions (e.g., the impact of bullying on suicide rates, or children or adults).
Delimiting factors could include:
- Research design : Mixed-methods research, including thematic analysis of semi-structured interviews and statistical analysis of a survey
- Timeframe : Data collection to run for 3 months
- Population size : 100 survey participants; 15 interviewees
- Recruitment of participants : Quota sampling (aiming for specific portions of men, women, ethnic minority students etc.)
We can see that every choice you make in planning and conducting your research inevitably excludes other possible options.
What's the difference between limitations and delimitations?
Delimitations and limitations are entirely different, although they often get mixed up. These are the main differences:
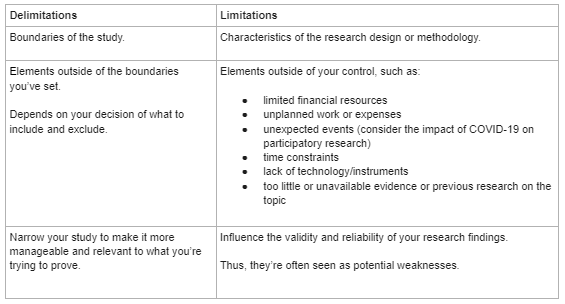
This chart explains the difference between delimitations and limitations. Delimitations are the boundaries of the study while the limitations are the characteristics of the research design or methodology.
Delimitations encompass the elements outside of the boundaries you've set and depends on your decision of what yo include and exclude. On the flip side, limitations are the elements outside of your control, such as:
- limited financial resources
- unplanned work or expenses
- unexpected events (for example, the COVID-19 pandemic)
- time constraints
- lack of technology/instruments
- unavailable evidence or previous research on the topic
Delimitations involve narrowing your study to make it more manageable and relevant to what you're trying to prove. Limitations influence the validity and reliability of your research findings. Limitations are seen as potential weaknesses in your research.
Example of the differences
To clarify these differences, go back to the limitations of the earlier example.
Limitations could comprise:
- Sample size : Not large enough to provide generalizable conclusions.
- Sampling approach : Non-probability sampling has increased bias risk. For instance, the researchers might not manage to capture the experiences of ethnic minority students.
- Methodological pitfalls : Research participants from an urban area (Paris) are likely to be more advantaged than students in rural areas. A study exploring the latter's experiences will probably yield very different findings.
Where do you write the scope and delimitations, and why?
It can be surprisingly empowering to realize you're restricted when conducting scholarly research. But this realization also makes writing up your research easier to grasp and makes it easier to see its limits and the expectations placed on it. Properly revealing this information serves your field and the greater scientific community.
Openly (but briefly) acknowledge the scope and delimitations of your study early on. The Abstract and Introduction sections are good places to set the parameters of your paper.
Next, discuss the scope and delimitations in greater detail in the Methods section. You'll need to do this to justify your methodological approach and data collection instruments, as well as analyses
At this point, spell out why these delimitations were set. What alternative options did you consider? Why did you reject alternatives? What could your study not address?
Let's say you're gathering data that can be derived from different but related experiments. You must convince the reader that the one you selected best suits your research question.
Finally, a solid paper will return to the scope and delimitations in the Findings or Discussion section. Doing so helps readers contextualize and interpret findings because the study's scope and methods influence the results.
For instance, agricultural field experiments carried out under irrigated conditions yield different results from experiments carried out without irrigation.
Being transparent about the scope and any outstanding issues increases your research's credibility and objectivity. It helps other researchers replicate your study and advance scientific understanding of the same topic (e.g., by adopting a different approach).
How do you write the scope and delimitations?
Define the scope and delimitations of your study before collecting data. This is critical. This step should be part of your research project planning.
Answering the following questions will help you address your scope and delimitations clearly and convincingly.
- What are your study's aims and objectives?
- Why did you carry out the study?
- What was the exact topic under investigation?
- Which factors and variables were included? And state why specific variables were omitted from the research scope.
- Who or what did the study explore? What was the target population?
- What was the study's location (geographical area) or setting (e.g., laboratory)?
- What was the timeframe within which you collected your data ?
- Consider a study exploring the differences between identical twins who were raised together versus identical twins who weren't. The data collection might span 5, 10, or more years.
- A study exploring a new immigration policy will cover the period since the policy came into effect and the present moment.
- How was the research conducted (research design)?
- Experimental research, qualitative, quantitative, or mixed-methods research, literature review, etc.
- What data collection tools and analysis techniques were used? e.g., If you chose quantitative methods, which statistical analysis techniques and software did you use?
- What did you find?
- What did you conclude?
Useful vocabulary for scope and delimitations
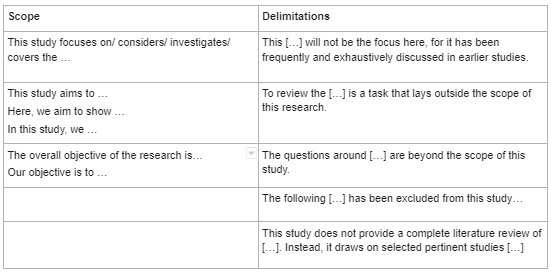
When explaining both the scope and delimitations, it's important to use the proper language to clearly state each.
For the scope , use the following language:
- This study focuses on/considers/investigates/covers the following:
- This study aims to . . . / Here, we aim to show . . . / In this study, we . . .
- The overall objective of the research is . . . / Our objective is to . . .
When stating the delimitations, use the following language:
- This [ . . . ] will not be the focus, for it has been frequently and exhaustively discusses in earlier studies.
- To review the [ . . . ] is a task that lies outside the scope of this study.
- The following [ . . . ] has been excluded from this study . . .
- This study does not provide a complete literature review of [ . . . ]. Instead, it draws on selected pertinent studies [ . . . ]
Analysis of a published scope
In one example, Simione and Gnagnarella (2020) compared the psychological and behavioral impact of COVID-19 on Italy's health workers and general population.
Here's a breakdown of the study's scope into smaller chunks and discussion of what works and why.
Also notable is that this study's delimitations include references to:
- Recruitment of participants: Convenience sampling
- Demographic characteristics of study participants: Age, sex, etc.
- Measurements methods: E.g., the death anxiety scale of the Existential Concerns Questionnaire (ECQ; van Bruggen et al., 2017) etc.
- Data analysis tool: The statistical software R
Analysis of published scope and delimitations
Scope of the study : Johnsson et al. (2019) explored the effect of in-hospital physiotherapy on postoperative physical capacity, physical activity, and lung function in patients who underwent lung cancer surgery.
The delimitations narrowed down the scope as follows:
Refine your scope, delimitations, and scientific English
English ability shouldn't limit how clear and impactful your research can be. Expert AJE editors are available to assess your science and polish your academic writing. See AJE services here .

The AJE Team
See our "Privacy Policy"

Community Blog
Keep up-to-date on postgraduate related issues with our quick reads written by students, postdocs, professors and industry leaders.
Scope and Delimitations – Explained & Example

- By DiscoverPhDs
- October 2, 2020

What Is Scope and Delimitation in Research?
The scope and delimitations of a thesis, dissertation or research paper define the topic and boundaries of the research problem to be investigated.
The scope details how in-depth your study is to explore the research question and the parameters in which it will operate in relation to the population and timeframe.
The delimitations of a study are the factors and variables not to be included in the investigation. In other words, they are the boundaries the researcher sets in terms of study duration, population size and type of participants, etc.
Difference Between Delimitations and Limitations
Delimitations refer to the boundaries of the research study, based on the researcher’s decision of what to include and what to exclude. They narrow your study to make it more manageable and relevant to what you are trying to prove.
Limitations relate to the validity and reliability of the study. They are characteristics of the research design or methodology that are out of your control but influence your research findings. Because of this, they determine the internal and external validity of your study and are considered potential weaknesses.
In other words, limitations are what the researcher cannot do (elements outside of their control) and delimitations are what the researcher will not do (elements outside of the boundaries they have set). Both are important because they help to put the research findings into context, and although they explain how the study is limited, they increase the credibility and validity of a research project.
Guidelines on How to Write a Scope
A good scope statement will answer the following six questions:

- Why – the general aims and objectives (purpose) of the research.
- What – the subject to be investigated, and the included variables.
- Where – the location or setting of the study, i.e. where the data will be gathered and to which entity the data will belong.
- When – the timeframe within which the data is to be collected.
- Who – the subject matter of the study and the population from which they will be selected. This population needs to be large enough to be able to make generalisations.
- How – how the research is to be conducted, including a description of the research design (e.g. whether it is experimental research, qualitative research or a case study), methodology, research tools and analysis techniques.
To make things as clear as possible, you should also state why specific variables were omitted from the research scope, and whether this was because it was a delimitation or a limitation. You should also explain why they could not be overcome with standard research methods backed up by scientific evidence.
How to Start Writing Your Study Scope
Use the below prompts as an effective way to start writing your scope:
- This study is to focus on…
- This study covers the…
- This study aims to…
Guidelines on How to Write Delimitations
Since the delimitation parameters are within the researcher’s control, readers need to know why they were set, what alternative options were available, and why these alternatives were rejected. For example, if you are collecting data that can be derived from three different but similar experiments, the reader needs to understand how and why you decided to select the one you have.
Your reasons should always be linked back to your research question, as all delimitations should result from trying to make your study more relevant to your scope. Therefore, the scope and delimitations are usually considered together when writing a paper.
How to Start Writing Your Study Delimitations
Use the below prompts as an effective way to start writing your study delimitations:
- This study does not cover…
- This study is limited to…
- The following has been excluded from this study…
Examples of Delimitation in Research
Examples of delimitations include:
- research objectives,
- research questions,
- research variables,
- target populations,
- statistical analysis techniques .
Examples of Limitations in Research
Examples of limitations include:
- Issues with sample and selection,
- Insufficient sample size, population traits or specific participants for statistical significance,
- Lack of previous research studies on the topic which has allowed for further analysis,
- Limitations in the technology/instruments used to collect your data,
- Limited financial resources and/or funding constraints.
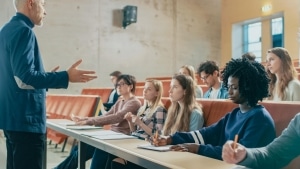
You’ll come across many academics with PhD, some using the title of Doctor and others using Professor. This blog post helps you understand the differences.

This post explains the difference between the journal paper status of In Review and Under Review.

If you’re about to sit your PhD viva, make sure you don’t miss out on these 5 great tips to help you prepare.
Join thousands of other students and stay up to date with the latest PhD programmes, funding opportunities and advice.
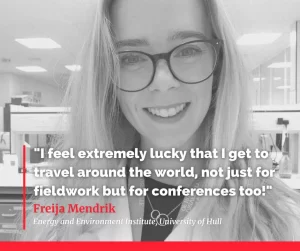
Browse PhDs Now

The Thurstone Scale is used to quantify the attitudes of people being surveyed, using a format of ‘agree-disagree’ statements.
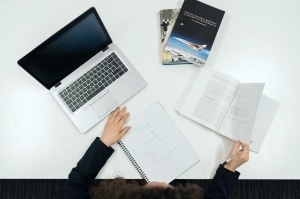
Do you need to have published papers to do a PhD? The simple answer is no but it could benefit your application if you can.

Kamal is a second year PhD student University of Toronto in the department of Chemistry. His research is focused on making hydrogen gas more affordable and easier to generate from water to use as a clean energy source.
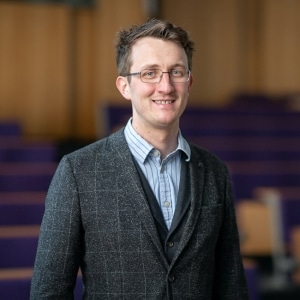
Dr Britton gained his DPhil in material science research at Oxford University in 2010. He is now a Senior Lecturer in Materials Science and Engineering at Imperial College London.
Join Thousands of Students
Academic Research in Education
- How to Find Books, Articles and eBooks
- Books, eBooks, & Multimedia
- Evaluating Information
- Deciding on a Topic
- Creating a Thesis Statement
- The Literature Review
- Scope of Research
Defining the Scope of your Project
What is scope.
- Choosing a Design
- Citing Sources & Avoiding Plagiarism
- Contact Library
Post-Grad Collective [PGC]. (2017, February 13). Thesis Writing-Narrow the Scope [Video file]. Retrieved from https://www.youtube.com/watch?v=IlCO5yRB9No&feature=youtu.be
Learn to cite a YouTube Video!
The scope of your project sets clear parameters for your research.
A scope statement will give basic information about the depth and breadth of the project. It tells your reader exactly what you want to find out , how you will conduct your study, the reports and deliverables that will be part of the outcome of the study, and the responsibilities of the researchers involved in the study. The extent of the scope will be a part of acknowledging any biases in the research project.
Defining the scope of a project:
- focuses your research goals
- clarifies the expectations for your research project
- helps you determine potential biases in your research methodology by acknowledging the limits of your research study
- identifies the limitations of your research
- << Previous: The Literature Review
- Next: Choosing a Design >>
- Last Updated: Mar 7, 2024 9:06 AM
- URL: https://moc.libguides.com/aca_res_edu

Faculty & Staff Directory
Event Calendar
News Archives
Privacy Policy
Terms & Conditions
Public Relations
634 Henderson St.
Mount Olive, NC 28365
1-800-653-0854

Setting Limits and Focusing Your Study: Exploring scope and delimitation
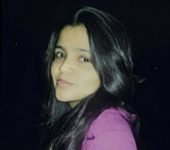
As a researcher, it can be easy to get lost in the vast expanse of information and data available. Thus, when starting a research project, one of the most important things to consider is the scope and delimitation of the study. Setting limits and focusing your study is essential to ensure that the research project is manageable, relevant, and able to produce useful results. In this article, we will explore the importance of setting limits and focusing your study through an in-depth analysis of scope and delimitation.
Company Name 123
Lorem ipsum dolor sit amet, cu usu cibo vituperata, id ius probo maiestatis inciderint, sit eu vide volutpat.
Sign Up for More Insights
Table of Contents
Scope and Delimitation – Definition and difference
Scope refers to the range of the research project and the study limitations set in place to define the boundaries of the project and delimitation refers to the specific aspects of the research project that the study will focus on.
In simpler words, scope is the breadth of your study, while delimitation is the depth of your study.
Scope and delimitation are both essential components of a research project, and they are often confused with one another. The scope defines the parameters of the study, while delimitation sets the boundaries within those parameters. The scope and delimitation of a study are usually established early on in the research process and guide the rest of the project.
Types of Scope and Delimitation
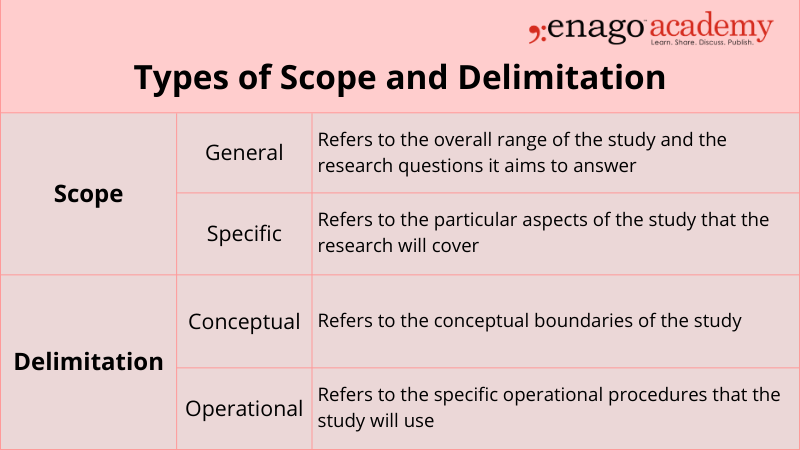
Significance of Scope and Delimitation
Setting limits and focusing your study through scope and delimitation is crucial for the following reasons:
- It allows researchers to define the research project’s boundaries, enabling them to focus on specific aspects of the project. This focus makes it easier to gather relevant data and avoid unnecessary information that might complicate the study’s results.
- Setting limits and focusing your study through scope and delimitation enables the researcher to stay within the parameters of the project’s resources.
- A well-defined scope and delimitation ensure that the research project can be completed within the available resources, such as time and budget, while still achieving the project’s objectives.
5 Steps to Setting Limits and Defining the Scope and Delimitation of Your Study
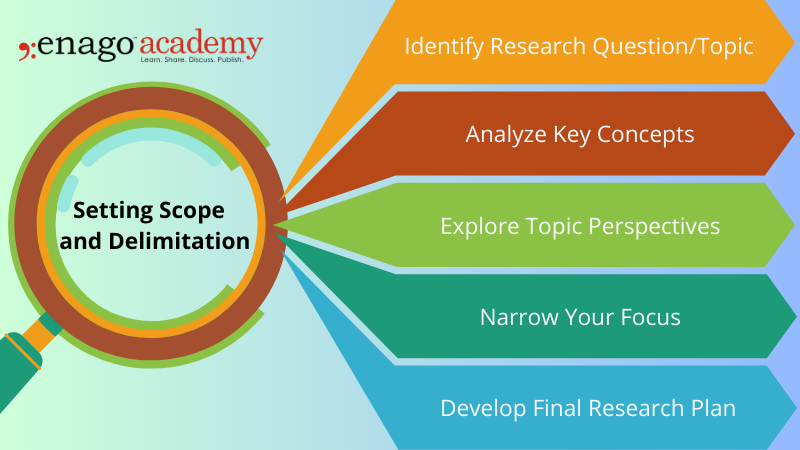
There are a few steps that you can take to set limits and focus your study.
1. Identify your research question or topic
The first step is to identify what you are interested in learning about. The research question should be specific, measurable, achievable, relevant, and time-bound (SMART). Once you have a research question or topic, you can start to narrow your focus.
2. Consider the key terms or concepts related to your topic
What are the important terms or concepts that you need to understand in order to answer your research question? Consider all available resources, such as time, budget, and data availability, when setting scope and delimitation.
The scope and delimitation should be established within the parameters of the available resources. Once you have identified the key terms or concepts, you can start to develop a glossary or list of definitions.
3. Consider the different perspectives on your topic
There are often different perspectives on any given topic. Get feedback on the proposed scope and delimitation. Advisors can provide guidance on the feasibility of the study and offer suggestions for improvement.
It is important to consider all of the different perspectives in order to get a well-rounded understanding of your topic.
4. Narrow your focus
Be specific and concise when setting scope and delimitation. The parameters of the study should be clearly defined to avoid ambiguity and ensure that the study is focused on relevant aspects of the research question.
This means deciding which aspects of your topic you will focus on and which aspects you will eliminate.
5. Develop the final research plan
Revisit and revise the scope and delimitation as needed. As the research project progresses, the scope and delimitation may need to be adjusted to ensure that the study remains focused on the research question and can produce useful results. This plan should include your research goals, methods, and timeline.
Examples of Scope and Delimitation
To better understand scope and delimitation, let us consider two examples of research questions and how scope and delimitation would apply to them.
Research question: What are the effects of social media on mental health?
Scope: The scope of the study will focus on the impact of social media on the mental health of young adults aged 18-24 in the United States.
Delimitation: The study will specifically examine the following aspects of social media: frequency of use, types of social media platforms used, and the impact of social media on self-esteem and body image.
Research question: What are the factors that influence employee job satisfaction in the healthcare industry?
Scope: The scope of the study will focus on employee job satisfaction in the healthcare industry in the United States.
Delimitation: The study will specifically examine the following factors that influence employee job satisfaction: salary, work-life balance, job security, and opportunities for career growth.
Setting limits and defining the scope and delimitation of a research study is essential to conducting effective research. By doing so, researchers can ensure that their study is focused, manageable, and feasible within the given time frame and resources. It can also help to identify areas that require further study, providing a foundation for future research.
So, the next time you embark on a research project, don’t forget to set clear limits and define the scope and delimitation of your study. It may seem like a tedious task, but it can ultimately lead to more meaningful and impactful research. And if you still can’t find a solution, reach out to Enago Academy using #AskEnago and tag @EnagoAcademy on Twitter , Facebook , and Quora .
Frequently Asked Questions
The scope in research refers to the boundaries and extent of a study, defining its specific objectives, target population, variables, methods, and limitations, which helps researchers focus and provide a clear understanding of what will be investigated.
Delimitation in research defines the specific boundaries and limitations of a study, such as geographical, temporal, or conceptual constraints, outlining what will be excluded or not within the scope of investigation, providing clarity and ensuring the study remains focused and manageable.
To write a scope; 1. Clearly define research objectives. 2. Identify specific research questions. 3. Determine the target population for the study. 4. Outline the variables to be investigated. 5. Establish limitations and constraints. 6. Set boundaries and extent of the investigation. 7. Ensure focus, clarity, and manageability. 8. Provide context for the research project.
To write delimitations; 1. Identify geographical boundaries or constraints. 2. Define the specific time period or timeframe of the study. 3. Specify the sample size or selection criteria. 4. Clarify any demographic limitations (e.g., age, gender, occupation). 5. Address any limitations related to data collection methods. 6. Consider limitations regarding the availability of resources or data. 7. Exclude specific variables or factors from the scope of the study. 8. Clearly state any conceptual boundaries or theoretical frameworks. 9. Acknowledge any potential biases or constraints in the research design. 10. Ensure that the delimitations provide a clear focus and scope for the study.

What is an example of delimitation of the study?
Thank you 💕
Thank You very simplified🩷
Thanks, I find this article very helpful
Rate this article Cancel Reply
Your email address will not be published.
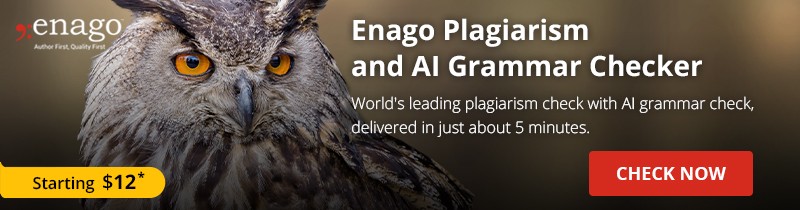
Enago Academy's Most Popular Articles
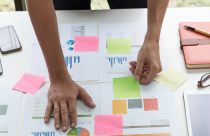
- Publishing Research
- Reporting Research
How to Optimize Your Research Process: A step-by-step guide
For researchers across disciplines, the path to uncovering novel findings and insights is often filled…
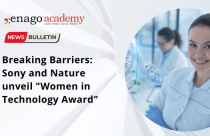
- Industry News
- Trending Now
Breaking Barriers: Sony and Nature unveil “Women in Technology Award”
Sony Group Corporation and the prestigious scientific journal Nature have collaborated to launch the inaugural…
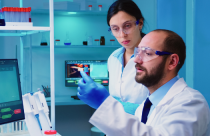
Achieving Research Excellence: Checklist for good research practices
Academia is built on the foundation of trustworthy and high-quality research, supported by the pillars…
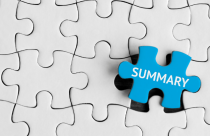
- Promoting Research
Plain Language Summary — Communicating your research to bridge the academic-lay gap
Science can be complex, but does that mean it should not be accessible to the…
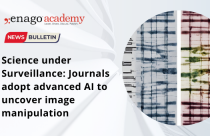
Science under Surveillance: Journals adopt advanced AI to uncover image manipulation
Journals are increasingly turning to cutting-edge AI tools to uncover deceitful images published in manuscripts.…
Choosing the Right Analytical Approach: Thematic analysis vs. content analysis for…
Research Recommendations – Guiding policy-makers for evidence-based decision making
Demystifying the Role of Confounding Variables in Research

Sign-up to read more
Subscribe for free to get unrestricted access to all our resources on research writing and academic publishing including:
- 2000+ blog articles
- 50+ Webinars
- 10+ Expert podcasts
- 50+ Infographics
- 10+ Checklists
- Research Guides
We hate spam too. We promise to protect your privacy and never spam you.
I am looking for Editing/ Proofreading services for my manuscript Tentative date of next journal submission:
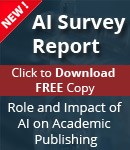
What should universities' stance be on AI tools in research and academic writing?
- Translators
- Graphic Designers
Please enter the email address you used for your account. Your sign in information will be sent to your email address after it has been verified.
Exploring Scope and Delimitation in Academic Research
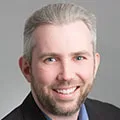
Academic research is a meticulous process that requires precise planning and clear boundaries. Two pivotal components in this process are the scope and delimitations of the study. The definitions and establishment of these parameters are instrumental in ensuring that the research is effective, manageable, and yields relevant results.
The "scope" of a research project refers to the areas that the study will cover. It is the breadth and depth of the investigation. It defines the subject matter, the geographical location, the time frame, and the issues that the study will explore. Essentially, the scope delineates what the researcher aims to cover in the study.
On the other hand, "delimitations" are the boundaries or limitations set by the researcher. They define what the study will not include. Delimitations could involve the choice of research methodology , the selection of respondents, the duration of the study, and more. They help in confining the study to a manageable size while excluding peripheral elements.
Understanding and correctly implementing scope and delimitations are vital to ensuring your research is well-defined and focused, facilitating higher accuracy and relevancy in your findings.
Importance of scope in research
"Scope" in research refers to the comprehensive extent of study—it outlines the parameters of what will be explored and addressed. It defines the topic of the research , the geographical region under study, the timeframe considered, and the issues that the study will address. The scope of a research project is vital because it determines the depth and breadth of your investigation.
Defining the scope of research is a fundamental step in the research process for several reasons. First, it provides a roadmap for the study, giving the researcher clear guidelines about what to include and exclude. Without a well-defined scope, research can become unmanageably vast or lose its focus.
Second, the scope ensures the research's relevance and applicability. It helps the researcher maintain a tight focus on the study's central question , ensuring that all aspects of the research contribute to answering this question. This focus aids in avoiding irrelevant diversions that could dilute the final conclusions.
Finally, a well-defined scope can help ensure the efficient use of resources. Research involves considerable time, effort, and often financial resources. By providing clear boundaries, the scope ensures these resources are utilized effectively without wasted effort on peripheral issues.
Suppose a research study is looking at the impacts of social media usage on mental health. If the scope is too broad—like examining all social media platforms' effects on all demographic groups worldwide—then the research can quickly become unwieldy and hard to manage. It would involve vast amounts of data, requiring considerable time, resources, and computational power to analyze effectively.
However, if the scope is narrowed down—such as investigating the impact of Instagram usage on the mental health of teenagers in a specific city over the past five years—the research becomes far more manageable. This specific focus allows for a more in-depth analysis and likely will provide more meaningful, actionable results. This example illustrates the importance of appropriately defining the scope of research for its successful execution.
Determining the scope of your research
Setting the scope of your research project is a critical and delicate task. Below are steps, tips, and common mistakes to avoid when determining the scope of your research:
Steps to define the scope
- Identify Your Topic: The first step involves identifying and understanding your research topic. This knowledge will serve as a basis for determining the breadth and depth of your study.
- Define Your Research Questions: The research questions are the heart of your study. They will help you determine the specific areas your research should cover.
- Establish Boundaries: Clearly establish the geographical, temporal, and topical boundaries of your research. These boundaries will guide the range of your study.
- Choose Your Methodology: Decide on the research methods you will use as these will directly impact the scope of your study.
Tips for a manageable scope
- Stay Focused: Stay concentrated on your research questions. Do not stray into areas that aren't directly relevant.
- Be Realistic: Consider the resources (time, money, manpower) available. Ensure your scope is feasible given these resources.
- Seek Guidance: Consult with your academic advisor or peers for feedback on your proposed scope.
Common mistakes to avoid
- Overly Broad Scope: Avoid setting an overly broad scope which could result in an unmanageable and unfocused study.
- Too Narrow Scope: Conversely, a scope that is too narrow may miss important aspects of the research topic.
- Ignoring Resources: Not taking into account available resources when setting the scope can lead to a project that is impossible to complete.
Defining the scope of your research is a delicate balance, requiring careful consideration of your research questions, resources, and the depth and breadth of investigation needed to answer these questions effectively.
Importance of delimitations in research
In the context of academic research, "delimitations" refers to the choices made by the researcher which define the boundaries of the study. These are the variables that lead the researcher to narrow the scope of the study from its potential vastness to a manageable size.
Delimitations might include the geographic area where the study is confined, the participants involved in the study, the methodology used, the time period considered, or the specific incidents or aspects the study will focus on. Essentially, delimitations are the self-imposed limitations on the scope of the study.
Defining the delimitations of a research project is crucial for several reasons. Firstly, they establish the context or setting in which the study occurs. This, in turn, allows for the work to be reproduced in a similar context for verification or refutation in future studies.
Secondly, delimitations provide a way to narrow the scope of the research to a manageable size, thus avoiding the pitfall of an overly ambitious project. They help researchers to stay focused on the main research questions and prevent diversion into irrelevant aspects.
Finally, clearly defined delimitations enhance the credibility of the research. They offer transparency about the research design and methodology, which adds to the validity of the results.
For instance, in a research study examining the impact of technology on student achievement in a certain district, examples of delimitations might include focusing only on public schools, considering only high school students, and confining the study to a particular school year. These choices help to focus the research and ensure its manageability. Therefore, delimitations play a pivotal role in structuring and guiding an effective and efficient research study.
Setting delimitations for your research
Establishing appropriate delimitations for your research project is an important part of research design. Here are some steps, guidelines, and common mistakes to consider when setting your research delimitations:
Steps to establish delimitations
- Identify the boundaries: Begin by deciding the geographical region, time period, and subject matter your research will cover.
- Determine Your Research Population: Identify the specific population your study will focus on. This could be based on age, profession, geographical location, etc.
- Choose Your Research Methods: Decide the specific methods you will use to collect and analyze data, as these decisions will also set limitations on your study.
Guidelines for choosing delimitations
- Align with Your Research Objectives: The delimitations should be in line with your research questions and objectives. They should help focus your study without detracting from its goals.
- Be Practical: Consider the resources available, including time, funds, and access to data. Your delimitations should be feasible given these constraints.
- Seek Input: Consult with your research advisor or peers. Their feedback can help ensure your delimitations are appropriate and well thought out.
Common errors to avoid:
- Unrealistic Delimitations: Be wary of setting delimitations that are too stringent or ambitious to be feasible given your resources and timeframe.
- Undefined Delimitations: Avoid leaving your delimitations vague or undefined. This can lead to scope creep, where your project expands beyond its initial plan, making it unmanageable.
- Ignoring Delimitations: Once set, stick to your delimitations. Deviating from them can lead to a loss of focus and can compromise the integrity of your results.
Setting delimitations is a crucial step in research planning. Properly defined delimitations can make your research project more manageable, maintain your focus, and ensure the effective use of your resources.
The interplay between scope and delimitations
The relationship between scope and delimitations in academic research is a dynamic and interdependent one. Each aspect serves to shape and refine the other, ultimately leading to a focused, feasible, and effective research design.
The scope of a research project describes the breadth and depth of the investigation—what it aims to cover and how far it intends to delve into the subject matter. The delimitations, on the other hand, identify the boundaries and constraints of the study—what it will not cover.
As such, the scope and delimitations of a research study are intimately connected. When the scope of a study is broad, the delimitations must be carefully considered to ensure the project remains manageable and focused. Conversely, when the scope is narrow, the delimitations might be less constraining, but they still play a critical role in defining the specificity of the research.
Balancing the scope and delimitations is crucial for an efficient research design. Too broad a scope without carefully defined delimitations can lead to a study that is unwieldy and lacks depth. On the other hand, a very narrow scope with overly rigid delimitations might result in a study that overlooks important aspects of the research topic.
Thus, researchers must strive to maintain a balance—establishing a scope that is wide enough to fully explore the research topic, but also setting appropriate delimitations to ensure the study remains feasible and focused. In doing so, the research will be well-structured and yield meaningful, relevant findings.
Role of scope and delimitations in research validity
Scope and delimitations are fundamental aspects of research design that directly influence the validity, reliability, and replicability of a study.
Research validity refers to the degree to which a study accurately reflects or measures the concept that the researcher intends to investigate. A well-defined scope is critical to research validity because it clearly delineates what the study will cover. This clear definition ensures that the research focuses on relevant aspects of the topic and that the findings accurately reflect the concept under investigation.
Similarly, carefully thought-out delimitations contribute to research validity by identifying what the study will not cover. This clarity helps to prevent the study from straying into irrelevant areas, ensuring that the research stays focused and relevant.
In addition to contributing to research validity, scope and delimitations also influence the reliability and replicability of a study. Reliability refers to the consistency of a study's results, while replicability refers to the ability of other researchers to repeat the study and obtain similar results.
A clearly defined scope makes a study more reliable by providing a detailed outline of the areas covered by the research. This clarity makes it more likely that the study will produce consistent results. Moreover, clearly defined delimitations enhance the replicability of a study by providing explicit boundaries for the research, which makes it easier for other researchers to repeat the study in a similar context.
In summary, a well-defined scope and carefully thought-out delimitations contribute significantly to the validity, reliability, and replicability of academic research. They ensure that the research is focused, that the findings are relevant and accurate, and that the study can be reliably repeated by other researchers.
Examples of scope and delimitation in well-known research
- The Milgram Experiment: Stanley Milgram's famous psychology experiment sought to understand obedience to authority figures. The scope of this study was clearly defined—it focused on how far individuals would go in obeying an instruction if it involved harming another person. However, delimitations were set to ensure manageability. Participants were delimited to male individuals, and the experiment was confined to a controlled laboratory setting. These delimitations allowed Milgram to manage the research effectively while maintaining the depth of his study on human behavior.
- The Framingham Heart Study: This ongoing cardiovascular study began in 1948 and is aimed at identifying common factors that contribute to cardiovascular disease. The scope of the research is broad, covering many aspects of lifestyle, medical history, and physical characteristics. However, the study set clear delimitations: it initially only involved adult residents of Framingham, Massachusetts. This geographical delimitation made this broad-scope study manageable and eventually yielded influential results that shaped our understanding of heart disease.
- The Marshmallow Test: This well-known study by Walter Mischel explored delayed gratification in children. The scope was clearly defined: the study aimed to understand the ability of children to delay gratification and how it related to future success. The delimitations of the study included the age of the participants (preschool children), the setting (a controlled experiment with a treat), and the measure of future success (academic achievement, ability to cope with stress, etc.). These delimitations helped keep the study focused and manageable.
In all these examples, the researchers set a clear scope to outline the focus of their studies and used delimitations to restrict the boundaries. This balance between scope and delimitation was key in conducting successful and influential research.
In academic research, defining the scope and delimitations is a pivotal step in designing a robust and effective study. The scope outlines the breadth and depth of the investigation, offering a clear direction for the research. Meanwhile, delimitations set the boundaries of the study, ensuring that the research remains focused and manageable. Together, they play a crucial role in enhancing the validity, reliability, and replicability of a study.
Understanding the interplay between scope and delimitations is key to conducting efficient research. A well-defined scope paired with thoughtfully set delimitations contribute to a study's feasibility and its potential to yield meaningful and applicable results. Mistakes in setting the scope and delimitations can lead to unwieldy, unfocused research or a study that overlooks important aspects of a research question.
Reviewing famous studies, like the Milgram Experiment, the Framingham Heart Study, and the Marshmallow Test, we observe how a balanced approach to setting scope and delimitations can result in influential and valuable findings. Therefore, researchers should give careful thought to defining the scope and delimitations of their studies, keeping in mind their research questions, available resources, and the need for balance between breadth and focus. By doing so, they pave the way for successful and impactful research outcomes.
Header image by Kübra Arslaner .
Related Posts
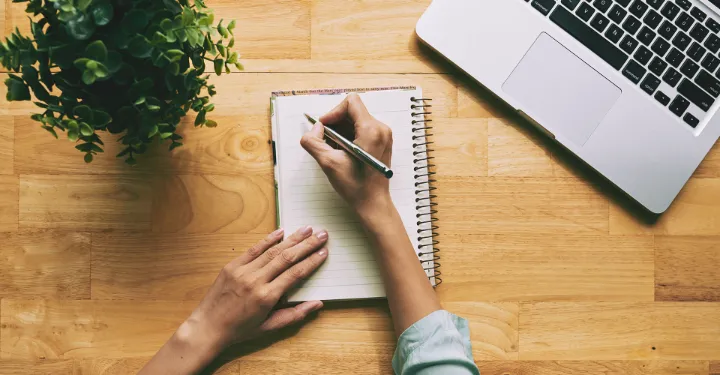
The Five Best Note-Taking Systems for College Students
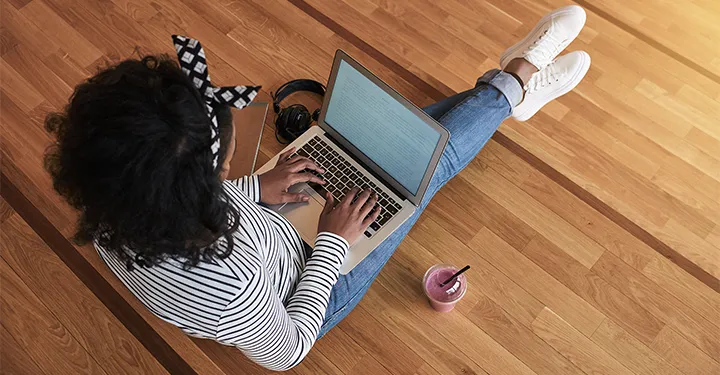
Tips for Writing an Effective Background of the Study
- Academic Writing Advice
- All Blog Posts
- Writing Advice
- Admissions Writing Advice
- Book Writing Advice
- Short Story Advice
- Employment Writing Advice
- Business Writing Advice
- Web Content Advice
- Article Writing Advice
- Magazine Writing Advice
- Grammar Advice
- Dialect Advice
- Editing Advice
- Freelance Advice
- Legal Writing Advice
- Poetry Advice
- Graphic Design Advice
- Logo Design Advice
- Translation Advice
- Blog Reviews
- Short Story Award Winners
- Scholarship Winners
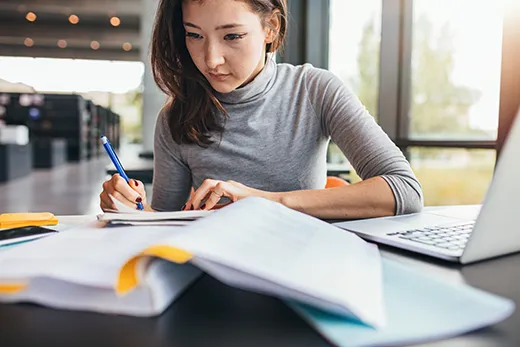
Need an academic editor before submitting your work?
Scope and Delimitations in Academic Research

Table of contents
- 1.1 Examples of Elements Included in the Scope
- 2.1 Examples of Delimitations in Research
- 3 Determining the Scope and Delimitation
- 4 Writing the Scope and Delimitations Section
- 5 Conclusion
Understanding the scope and delimitations of a study is crucial for defining its parameters and ensuring focused research efforts. What are delimitations in a research study? These components establish the boundaries within which the research will operate and clarify what the study aims to explore and achieve. This article delves into the significance of clearly defining the scope and every delimitation, how they guide the research focus, and their roles in shaping the research process. Additionally, it provides insights into determining these aspects and articulating them effectively in a research proposal or paper. Transitioning smoothly into the main discussion, let’s explore the importance of scope in research, guiding the focus.
The importance of Clearly Defining the Scope of the Study for Guiding Research Focus
The scope of research delineates its extent or range of inquiry, setting clear parameters for what the study will cover. It’s a foundational aspect that guides every step of the research process, from the formulation of research questions to the interpretation of results. Defining the scope helps in focusing the research efforts, ensuring that the study remains manageable and within realistic bounds.
Understanding the scope and limitation of the study allows researchers to allocate resources efficiently, ensuring that every aspect of the study receives adequate attention. It also helps in avoiding the common pitfall of overreaching, which can dilute the research’s impact and make findings less actionable. By setting a defined scope, researchers can more easily communicate their work’s relevance, limitations and delimitations in the research process to stakeholders, enhancing the credibility and applicability of their findings. Furthermore, a well-defined scope can facilitate a more targeted and effective literature review, laying a solid foundation for the research study.
When navigating the complexities of defining a study’s scope, researchers might seek external support to ensure their research is concise, well-structured, and impactful. A writing service , PapersOwl offers a spectrum tailored to meet academic research’s unique demands. Their expertise can be particularly beneficial in refining research proposals, ensuring the scope is clearly communicated and aligned with academic standards. Engaging with such a service allows researchers to benefit from professional insights, which can enhance the coherence and focus of their work. This collaboration can be instrumental in identifying the most relevant study areas and avoiding unnecessary diversions. With PapersOwl’s support, researchers can ensure their project’s scope is well-defined and compellingly presented, making a strong case for its significance and feasibility. This partnership can be a strategic step towards achieving a study’s specific objectives, ensuring it contributes valuable insights within its defined boundaries.
Examples of Elements Included in the Scope
Defining the scope of a research project is akin to drawing a map for a journey; it outlines the terrain to be explored and the boundaries within which the exploration will occur. This clarity is essential for guiding the research process, ensuring the investigation remains focused and relevant. The scope encompasses various elements, each contributing to the overall direction and integrity of the study. Let’s delve into some of these key elements:
- Research Objectives : The specific aim the study is designed to achieve.
- Geographical Coverage: The physical or virtual locations where the research is conducted.
- Time Frame: The period during which the study takes place, which could range from a few days to several years.
- Subject: The specific topics or issues the research intends to address.
- Population Being Studied: The group of individuals, organizations, or phenomena being investigated.
These components of the scope serve as critical navigational tools in the research journey. They ensure that the study remains grounded in its objectives, relevant to its intended audience or population, and manageable within its temporal and geographical constraints. By carefully defining these elements at the outset, researchers can avoid common pitfalls such as scope creep, where the study’s focus broadens uncontrollably, potentially diluting its impact and significance. A well-defined scope is instrumental in crafting a focused, coherent, and impactful research project.
Role of Delimitations in Qualitative Research
Delimitations in research examples specify the boundaries set by the investigator on what the study will not cover, distinguishing them from limitations, which are potential weaknesses in the study not controlled by the researcher. Delimitations are choices made to narrow the scope of a study, focusing on specific aspects while excluding others. In the intricate tapestry of research design, delimitations play a pivotal role in sharpening the focus and enhancing the clarity of a study. By explicitly stating what the research will not explore, delimitations help prevent the dispersion of the research efforts across too broad an area, thereby increasing the depth and specificity of the investigation. This strategic narrowing allows researchers to concentrate their inquiries on areas most likely to yield impactful insights, making efficient use of available resources and time.
One might wonder how to establish these boundaries effectively without compromising the potential breadth of discovery. Here, the expertise provided by platforms like PapersOwl, particularly their research paper help service, becomes invaluable. Their seasoned professionals can offer guidance on crafting a research design that is both focused and flexible, assisting in identifying and justifying delimitations that enhance the study’s relevance and feasibility. Through such collaboration, researchers can balance the scope and delimitation of the study, ensuring that it remains grounded in its objectives while open to unforeseen insights.
Furthermore, acknowledging delimitations in a research paper demonstrates a researcher’s critical understanding of their study’s context and constraints, enhancing the credibility of their work. It shows a mindful engagement with the research process, recognizing that by setting deliberate boundaries, the study can delve more deeply and meaningfully into its chosen area of inquiry. Thus, when thoughtfully articulated with support from research paper writing help, like that offered by PapersOwl, delimitation in research becomes a testament to the rigor and integrity of its effort.
Examples of Delimitations in Research
Delimitations in research are akin to the guardrails on a highway; they keep the investigation on track and prevent it from veering into less relevant or overly broad territories. Below are some examples of how researchers can apply delimitations to fine-tune their investigations:
- Restricting the Study to Certain Age Groups: Focusing on a specific demographic, such as teenagers or the elderly.
- Geographic Locations: Limiting the research to a particular country, city, or region.
- Specific Periods: Studying a phenomenon during a particular time frame, ignoring other periods.
Setting these research delimitations is not about narrowing the vision of the research, but rather about sharpening its focus. It allows for a more thorough and nuanced exploration of the chosen subjects, leading to more precise findings and general delimitation meaning in research. Delimitations highlight the researcher’s awareness of the study’s scope and commitment to conducting a focused, manageable investigation.
Determining the Scope and Delimitation
Identifying the scope and delimitations of your research involves understanding the research problem deeply and recognizing what is feasible within the constraints of time, resources, and data availability. Strategies for determining these include:
- Reviewing existing literature to identify gaps and opportunities.
- Consulting with experts or advisors to refine research questions.
- Considering data availability and methodological constraints.
Balancing the scope and delimitations involves ensuring the research is neither too broad, unmanageable, nor too narrow, limiting its significance. Crafting a research project that strikes the right balance between breadth and depth is a nuanced task. It requires a researcher to be acutely aware of where their study begins and ends, what it encompasses, and what it intentionally leaves out. This equilibrium is not found in isolation but through a diligent exploration of the field and an understanding of how to best position one’s work within it. A key step in this process is identifying and sourcing relevant literature and data, which can significantly influence the scope of research.
Leveraging resources such as PapersOwl’s guide on how to find sources for research papers can prove invaluable in this phase. This platform provides insights into locating credible and relevant information, ensuring that researchers build their work upon a solid foundation of existing knowledge. By understanding how to navigate the vast, effective ocean of available data, researchers can make informed decisions about the direction and limits of their study. This meticulous preparation is crucial for defining the scope and delimitations and justifying them within the context of the research proposal or paper. It demonstrates a researcher’s commitment to rigor and depth, showing that their choices are informed by a comprehensive understanding of the subject and its existing body of literature.
Writing the Scope and Delimitations Section

Articulating the scope and delimitations in a research paper or proposal is crucial for setting clear expectations. It should clearly define delimitations and what the study will and will not cover, providing a rationale for these choices. Effective wording and structure involve:
- Stating the research objectives and questions upfront.
- Describing the research methodology , data collection methods and analysis.
- Outlining the geographical coverage, time frame, and subject matter.
- Clearly stating the delimitations and the reasons behind them.
The presentation of the scope and delimitations within a research document not only guides the readers through the intentions of the research but also establishes a framework for evaluating the findings. It’s a critical section where transparency and precision are paramount, allowing the audience to grasp the extent of the study and the rationale behind its boundaries. This transparency is essential for the credibility of the research, as it demonstrates a conscious and deliberate effort to focus the investigation and acknowledges the existence of boundaries that the study does not cross.
To ensure clarity and impact, this section should seamlessly integrate with the overall narrative of the research proposal or paper. Researchers are advised to avoid jargon and overly technical language, making the research scope and delimitations accessible to a broader audience. This includes a layperson who may not have deep expertise in the field but an interest in the study’s outcomes. Additionally, it is beneficial to highlight how the defined study scope and delimitations contribute to addressing the research problem, filling knowledge gaps, or exploring uncharted territories.
Moreover, this part of the document offers an opportunity to discuss how the chosen delimitations enhance the study’s focus and depth. By justifying the exclusions, researchers can address potential critiques head-on, reinforcing the methodological choices and underscoring the study’s contribution to the field. This careful articulation ensures that the research is perceived as a well-thought-out endeavor, grounded in a strategic approach to inquiry.
The scope and delimitations of a study are foundational elements that guide the research process, setting clear boundaries and focusing efforts. By defining these aspects clearly, researchers can provide a clear roadmap for their investigation, ensuring that their work is both manageable and relevant. By consciously deciding what to exclude from the study, researchers can intensify their focus on the chosen subject, ensuring that the research efforts are concentrated where they are most needed and can be most effective. These self-imposed boundaries are critical for maintaining the study’s coherence and depth. This clarity not only aids in conducting the research but also in effectively communicating its implications, limits, and outcomes.
Readers also enjoyed
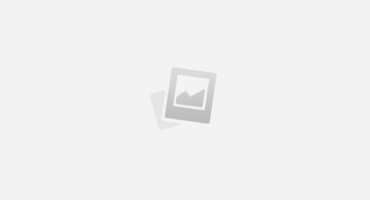
WHY WAIT? PLACE AN ORDER RIGHT NOW!
Just fill out the form, press the button, and have no worries!
We use cookies to give you the best experience possible. By continuing we’ll assume you board with our cookie policy.

How to write the scope of the study?
The scope of the study refers to the elements that will be covered in a research project. It defines the boundaries of the research. The scope is always decided in the preliminary stages of a study. Deciding it in the later stages creates a lot of ambiguity regarding the research goals. The main purpose of the scope of the study is that explains the extent to which the research area will be explored and thus specifies the parameters that will be observed within the study. In other words, it enables the researcher to define what the study will cover and the elements that it will not. Defining the scope helps the researcher acquire a high level of research and writing capability.
Goals of establishing the scope of the study
The following steps can help the researcher to effectively define the goals of establishing a scope of the study.
Identification of the project or research needs
The first step is to identify the research needs. This helps them set a benchmark from the first step. Identification of the ‘what’ and ‘why’ enables the researcher to clearly set the research goals and objectives and the manner in which they will be performed.
Confirmation of the goals and objectives of the research
The goals and objectives defined in the project scope should be aligned with the SMART (Specific, Measurable, Achievable, Realistic and Timeframe) guidelines, which are:
- Specific- this involves a clear specification of what the researcher wants to achieve. It involves specifying what, why and how things will be done. This reduces the chances of ambiguities and any misunderstanding in the future.
- Measurable- Goals should be measurable and dynamic so that constant feedback can be generated for improvement.
- Achievable- Research goals should be achievable with the resources that are available.
- Realistic- Goals should be easier to deliver so that complications that can hamper the quality of the research can be avoided. Other considerations to be kept in mind are the budget and timeline.
- Time frame- lastly, the researcher should estimate whether the set goals can be achieved within the given time frame or not.
Expectations and Acceptance
The researcher should take into account the expectations of the research and how well the findings of the researcher will be accepted by the reader. For instance, will the findings of your study help in policymaking or not?
Identification of the constraints
there are always certain roadblocks in conducting research, such as environmental conditions, technological inefficiency and lack of resources. Identifying these limitations and their possible solutions in advance help achieve goals better.
Identifying the necessary changes
After the preliminary goals are set, the researcher must carry out some part of the research so that necessary changes that lead to waste of time and resources at later stages are reduced. For example, while conducting an interview, if the researcher believes that the sample size decided is too large or too small according to the scope of the study, then the researcher can make the necessary changes in that order to avoid wastage of time and resources.
Guidelines for writing the scope of the study
The major things that the researcher should keep in mind while writing the scope of the study are as follows.
- Time period: While writing the scope of the study the researcher should first mention or state categorically the time periods the study will cover. Generally, the researchers combine the scope of the study with the limitation of the study. These things are quite interwoven. The main difference between the two is that limitations further cover the points like monetary constraints or non-cooperation from the side of the target audience.
- Geography: In addition to this another major point that the researcher should keep in mind is that the scope of the study should state the specific aspect of the data that needs to be collected like the geographic locations and the variables.
- Research population: Another major aspect that should be involved while writing the scope of the study is the sample size or the population that the researcher has selected for the study. The sampling plan must clearly indicate the sample universe, target population, profile and sample size with justification.
- Theories: The researcher should state the academic theories that are being applied to the data collected so that the reader better knows the lens of the analysis. This is presented in the ‘theoretical framework’ section.
- Purpose: The scope of the study must indicate the purpose behind it. It must briefly define the larger picture, i.e. the overall goal the researcher is trying to achieve.
- Limitations: It is impossible to avoid roadblocks in research. Every research is restricted in scope and is subjected to certain limitations. By acknowledging these limitations and how they are restricting the study makes its findings even more credible.

Consider the topic ‘Analysis of the role of social media on the educational development in India from 2000-2015’. The scope of the study for this research topic should include several roles within the mentioned time period. Further, it should also cover the mass media types that have been used in the analysis of the study also including the location and the sample size as well.
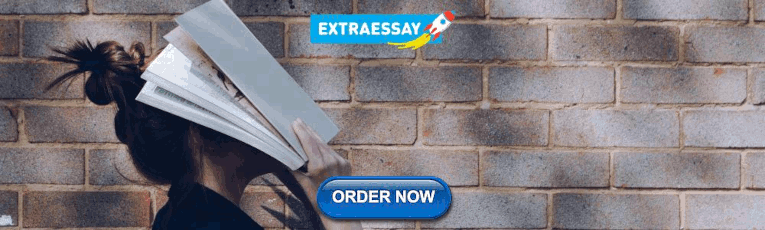
Scope of the study
With the increase in the number of social media users and its use in everyday communication at the individual and organizational levels, there has been a corresponding increase in its incorporation in educational development and especially in a country like India. In view of this situation, the present study analyzes the role of social media on the educational development of students. To this end, the study will also cover the changes in the usage of social media in the educational field over the time period ranging from 2000-2015. The scope of the study is restricted to select social media platforms, specifically Facebook, Twitter and YouTube. The empirical study in this research is restricted to five universities located across India, wherein the opinions of 30 teachers were studied in interview sessions. Further, the study also involves an analysis of students’ perspectives on the role of social media in education from the same university. Therefore the scope of this study is limited to India, and more specifically to those offering Arts and Science-related courses.
- Click to share on Twitter (Opens in new window)
- Click to share on Facebook (Opens in new window)
- Click to share on LinkedIn (Opens in new window)
- Click to share on WhatsApp (Opens in new window)
- Click to share on Telegram (Opens in new window)
Notify me of follow-up comments by email.
91 thoughts on “How to write the scope of the study?”
Proofreading.
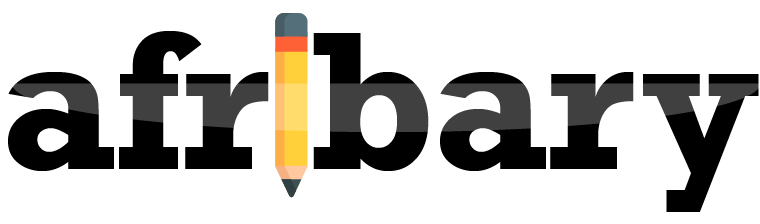
What is the Scope of the Study in Research?
The scope of the study explains the extent to which your research area will be explored, and the parameters the study will operate. It gives the reader and the writer an insight into what the study is aimed at and what should be anticipated.
This implies that the scope of the study should define the purpose of your study, the sample size and qualities, geographical location, the timeframe at which the study will be executed, theories the study will focus on, etc.
The scope of the study is just an aspect of research writing, and great attention needs to be taken not to go beyond what is expected. Therefore, the scope of the study sheds light on areas your study will cover and what it focuses on. What your study area is not going to focus on is of no relevance to your research study, and the scope of the study eliminates that.
Thank you for visiting nature.com. You are using a browser version with limited support for CSS. To obtain the best experience, we recommend you use a more up to date browser (or turn off compatibility mode in Internet Explorer). In the meantime, to ensure continued support, we are displaying the site without styles and JavaScript.
- View all journals
- My Account Login
- Explore content
- About the journal
- Publish with us
- Sign up for alerts
- Open access
- Published: 17 April 2024
Cross-link assisted spatial proteomics to map sub-organelle proteomes and membrane protein topologies
- Ying Zhu ORCID: orcid.org/0000-0003-4158-8093 1 ,
- Kerem Can Akkaya ORCID: orcid.org/0000-0002-6214-2229 1 , 2 ,
- Julia Ruta ORCID: orcid.org/0009-0007-0524-6885 1 ,
- Nanako Yokoyama 1 ,
- Cong Wang 1 ,
- Max Ruwolt ORCID: orcid.org/0009-0000-7220-406X 1 ,
- Diogo Borges Lima 1 ,
- Martin Lehmann ORCID: orcid.org/0000-0002-8370-6353 2 &
- Fan Liu ORCID: orcid.org/0000-0002-2358-549X 1 , 3
Nature Communications volume 15 , Article number: 3290 ( 2024 ) Cite this article
3272 Accesses
21 Altmetric
Metrics details
- Mass spectrometry
- Membrane proteins
- Mitochondria
- Protein–protein interaction networks
The functions of cellular organelles and sub-compartments depend on their protein content, which can be characterized by spatial proteomics approaches. However, many spatial proteomics methods are limited in their ability to resolve organellar sub-compartments, profile multiple sub-compartments in parallel, and/or characterize membrane-associated proteomes. Here, we develop a cross-link assisted spatial proteomics (CLASP) strategy that addresses these shortcomings. Using human mitochondria as a model system, we show that CLASP can elucidate spatial proteomes of all mitochondrial sub-compartments and provide topological insight into the mitochondrial membrane proteome. Biochemical and imaging-based follow-up studies confirm that CLASP allows discovering mitochondria-associated proteins and revising previous protein sub-compartment localization and membrane topology data. We also validate the CLASP concept in synaptic vesicles, demonstrating its applicability to different sub-cellular compartments. This study extends the scope of cross-linking mass spectrometry beyond protein structure and interaction analysis towards spatial proteomics, and establishes a method for concomitant profiling of sub-organelle and membrane proteomes.
Similar content being viewed by others
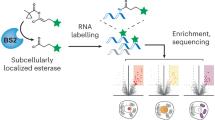
Bioorthogonal masked acylating agents for proximity-dependent RNA labelling
Shubhashree Pani, Tian Qiu, … Bryan C. Dickinson
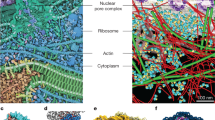
Bridging structural and cell biology with cryo-electron microscopy
Eva Nogales & Julia Mahamid
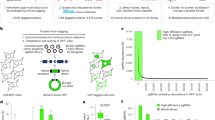
Pooled multicolour tagging for visualizing subcellular protein dynamics
Andreas Reicher, Jiří Reiniš, … Stefan Kubicek
Introduction
Cellular processes are mediated through complex interactions of biological molecules. To precisely control these interactions, cells are compartmentalized into various membrane-bound and membrane-less compartments that carry out specialized functions. Understanding the molecular basis of compartment-specific cellular functions requires insights into the spatial distribution of proteins and their dynamics. To enable protein localization profiling with high throughput and in a system-wide manner, various liquid chromatography mass spectrometry (LC-MS)-based spatial proteomics methods have been developed 1 , 2 , including Dynamic Organellar Maps (DOMs) 3 , Localisation of Organelle Proteins by Isotope Tagging (LOPIT) 4 , and Protein Correlation Profiling 5 , 6 . However, these methods depend on subcellular fractionation, which limits their spatial resolution because separation of different organelles is often incomplete and organelle sub-compartments cannot be resolved.
Information on sub-compartment-specific protein localization can be obtained by proximity-dependent enzymatic labeling approaches such as APEX, BioID and similar strategies (TurboID, APEX2, etc.) 7 , 8 . These methods (referred to as APEX/BioID for the remainder of the paper) rely on fusing a biotinylating enzyme to a protein of known localization or a peptide sequence targeted to a specific sub-compartment, enabling the enzyme-assisted biotin labeling of proximal proteins. Therefore, APEX/BioID methods require multiple experiments to capture different sub-compartments and applying them to characterize membrane-associated proteomes remains challenging. Furthermore, their labeling radius is difficult to control 9 , compromising the spatial resolution. In addition, the required engineering and ectopic expression of the target protein/peptide may introduce artifacts.
A potential yet unexplored alternative to fractionation- and proximity labeling-based spatial proteomics methods is cross-linking mass spectrometry (XL-MS) 10 . In XL-MS, proteins are covalently linked using small organic molecules (cross-linkers) composed of a spacer arm and two functional groups that are reactive toward specific residues. Subsequently, LC-MS is used to identify residue-to-residue cross-links. A cross-link can only occur if the distance between two residues is small enough to be bridged by the cross-linker. Consequently, the radius of XL-MS is clearly defined by the spacer arm length of the selected cross-linker 11 , which is typically 5–20 Å (0.5–2 nm). This suggests that XL-MS may enable spatial proteome profiling at a higher and more easily controllable spatial resolution than BioID (ca. 10 nm 12 ), APEX (ca. 20 nm 13 and 269 ± 41 nm 9 ), and µMap-based labeling (ca. 4 nm 14 and 54 ± 12 nm 9 , currently only applicable to cell surface proteins). However, even though we and others have developed methods for proteome-wide XL-MS 10 , 15 , 16 and have shown that these approaches can capture large parts of the proteome in intact cells and organelles 17 , 18 , 19 , 20 , 21 , 22 , 23 , cross-linking has so far only been used to analyze protein structures and interactions.
Here, we demonstrate that, beyond its utility in structural biology and interactomics, cross-linking enables high-resolution systematic mapping of protein localizations and membrane protein topologies. We establish the concept of cross-link assisted spatial proteomics (CLASP) by analyzing intact human mitochondria cross-linked with the commonly used disuccinimidyl sulfoxide (DSSO) 24 reagent. Mitochondria of cultured human cells are a popular model system for establishing spatial proteomics methods 25 , 26 , 27 because they consist of multiple spatially distinct sub-compartments - the outer membrane (OMM), the intermembrane space (IMS), the inner membrane (IMM), and the matrix 28 . They have been extensively characterized by APEX/BioID methods 26 , 27 , 29 , 30 , which are the only other approaches that can yield sub-compartment spatial information and insights into membrane protein topology. These published APEX/BioID datasets can serve as a performance benchmark for CLASP. Collectively, the mitochondrial model system offers architectural features and published data that allow us to evaluate whether CLASP can (1) determine protein localization with sub-compartment resolution, (2) characterize several sub-compartments in parallel, (3) capture membrane protein topology, and (4) add value compared to existing spatial proteomics approaches. To show that CLASP is broadly valid and utilizable, we apply it to another human mitochondria dataset and to a dataset obtained with synaptic vesicles (SVs) from mouse brain, both generated using the enrichable azide-tagged, acid-cleavable disuccinimidyl bis-sulfoxide (hereafter referred to as DSBSO) 31 cross-linker. While the CLASP analysis of DSBSO-cross-linked SVs serves as an additional proof of concept, CLASP of DSBSO-cross-linked mitochondria deepens the coverage of the mitochondrial interactome, allowing us to confirm most of the spatial annotations from our original DSSO-based CLASP analysis and add more localization and topology predictions. We verify several of our findings through biochemical, imaging and bioinformatics approaches, demonstrating the effectiveness and robustness of CLASP for elucidating protein localizations with high spatial resolution.
Proteome-wide XL-MS can reveal a high number of inter-protein connections
CLASP is based on the idea that system-wide XL-MS experiments are very likely to capture some well-characterized proteins with known subcellular localization, since such localization markers (LMs) tend to be highly abundant. The cross-links of these LMs allow deducing the relative localization of the directly connected proteins. CLASP analysis can thus extract spatial information from any XL-MS dataset of a biological system, provided that (1) a substantial number of inter-protein cross-links is detected, (2) cross-links are formed with a defined labeling radius, and (3) some LMs are captured. To assess whether these three conditions are fulfilled in a standard proteome-wide XL-MS experiment, we analyzed intact mitochondria isolated from HEK293T cells using DSSO cross-linker in three biological replicates (Fig. 1A , Supplementary Fig. 1 ). We identified 13971 unique cross-links from 1451 proteins by imposing a 2% FDR at the level of unique cross-linked residue pairs separately for intra-protein and inter-protein links. This dataset includes 6250 intra-protein and 7721 inter-protein cross-links corresponding to 2606 protein-protein connections (Supplementary Data 1 – 4 ), confirming that our XL-MS dataset meets the first requirement for CLASP.
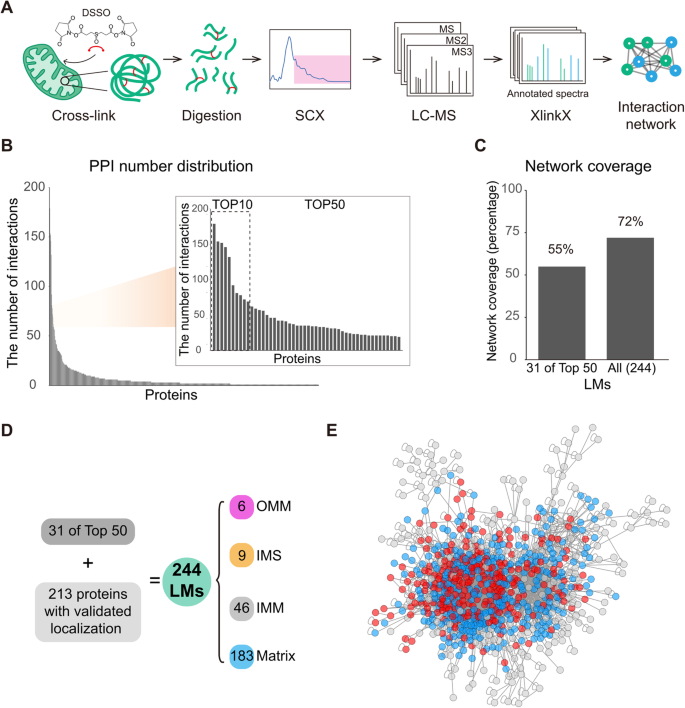
A Workflow for the XL-MS analysis of human mitochondrial proteins. B All identified proteins ranked by the number of their interactions. The inset shows the top 10 and top 50 most-connected proteins. The top 10 proteins are HSPD1, MDH2, HSPE1, ATP5F1A, HSPA9, C1QBP, CYCS, ATP5F1B, PHB2, and SHMT2. C Network coverage achieved when considering the first-tier interactors of 31 LMs derived from the top 50 most-connected proteins (left bar) and all LMs (right bar). D The origins and sub-compartment localizations for all 244 LMs. Proteins were selected as LMs if (i) their sub-mitochondrial localization had been thoroughly established in previous work, (ii) they were part of the top 50 most-connected proteins, had a corresponding PDB structure, or were a component of a well-studied mitochondrial protein assembly, and (iii) there are no cross-links contradicting their sub-compartment localization. E Overview of the mitochondrial PPI network, showing that XL-MS achieves a high degree of interconnectivity. The majority of the network is covered by LMs (red) and their first-tier interactors (blue), i.e. the proteins considered in the CLASP analysis. Source data are provided as a Source Data file.
Cross-linking provides a well-defined maximum labeling radius
To test whether our dataset also meets the second criterion, we set out to define the labeling radius of the applied DSSO cross-linker. It has a spacer arm of 10.3 Å and connects lysine residues, which have a side chain length of 7.6 Å. Taking into account a protein in-solution flexibility of 10 Å, we hypothesized that the maximum labeling radius in our CLASP experiment (defined as the C α -C α distance between two cross-linked lysines) will be < 4 nm/40 Å.
Traditional XL-MS studies have firmly established that the maximum cross-linked C α -C α distances are in good agreement with high-resolution protein structures. The cross-links in our mitochondrial dataset confirm this: We mapped 483 cross-links (357 intra-protein links and 126 inter-protein links) on high-resolution structures of 31 mitochondrial protein complexes and found that 96% of the measured Cα-Cα distances agree with the 4 nm distance constraint (Supplementary Figs. 2A and S3 ). However, this type of validation is limited to structurally characterized protein complexes, still raising the question whether the CLASP labeling radius is equally well controlled for the remaining proteins.
To begin to address this point, we took advantage of existing knowledge on the mitochondrial ultrastructure. Comparing our XL-based network to sub-cellular localization information from Swiss-Prot shows that we detected proteins in all mitochondrial sub-compartments, proving that the cross-linker penetrated both the OMM and the IMM. The distance between OMM and IMM is 20 nm as previously shown by electron tomography 28 . Therefore, an OMM protein should only cross-link to an IMM protein if their IMS regions are long enough to reduce the distance between their lysines to <4 nm. We analyzed this possibility for the only two OMM-IMM cross-links we detected between LMs: ATAD3A-TOMM20 and ATAD3A-TOMM40. Considering the linear length of their IMS regions (calculated as 3.5 Å per amino acid * number of amino acids), both cross-linked lysine pairs fall into the permitted distance (Supplementary Fig. 2B ). Notably, we do not observe OMM-IMM cross-links between proteins whose IMS protrusions are too short to achieve a mutual distance of 4 nm or lower.
As another line of evidence, we analyzed cross-links involving mitochondrial membrane proteins of known topology. Although DSSO penetrates both OMM and IMM, we should not observe cross-links across these membranes because their thickness is about 7 nm 32 , exceeding the assumed maximum labeling radius for DSSO. In agreement with this assumption, we do not detect any cross-links across the OMM and IMM, i.e. all our cross-linked amino acid pairs are localized within the same membrane-bound sub-compartment. To illustrate this, we show that the IMM-embedded proteins of the mitochondrial respiratory chain are exclusively involved in membrane-separated, sub-compartment-specific cross-links (Supplementary Fig. 2C ). This finding provides further evidence that the DSSO labeling distance is well-controlled and does not exceed 4 nm, thus fulfilling the second requirement for CLASP.
XL-MS captures reliable localization markers for CLASP-based spatial predictions
To evaluate whether our standard XL-MS experiment also fulfilled the third prerequisite for CLASP – the detection of robust LMs – we first filtered our XL-MS dataset by removing proteins lacking inter-protein links or forming small disconnected clusters resulting in an interactome of 2523 protein-protein connections among 748 proteins. Since these proteins are part of a well-connected interactome and thus in direct contact to other mitochondrial or mitochondria-associated proteins, they can be assigned to this organelle with reasonable confidence. To put this 748-protein dataset into context, we compared it to published mitochondrial proteome resources. In particular, we considered the MitoCarta3.0 database 33 , the MitoCoP database generated by combining different proteomics approaches 34 and mitochondrial datasets generated by DOMs 35 , LOPIT-DC and HyperLOPIT 36 (Supplementary Fig. 4 ). This analysis reveals a core of 286 proteins, which appear in the DSSO XL-MS data as well as all published datasets and databases. It also shows that 73% of the mitochondrial proteins found by DSSO cross-linking have been assigned to this organelle by at least one other resource. This overlap is similar to the results of the DOMs dataset, which show a 72% agreement with other resources.
To identify LMs in this DSSO-cross-linking-based mitochondrial interactome, we assessed the protein connectivity (Fig. 1B ). We found that 40 of the 50 most connected proteins have well-established sub-mitochondrial localizations. Since our goal is to use CLASP for making biological discoveries with the highest possible confidence, we sought to maximize the number of reliable LMs in our mitochondrial protein network by also including several well-characterized mitochondrial proteins and complexes such as VDAC, TIM/TOM complex, mitochondrial contact site and cristae organizing system (MICOS), oxidative phosphorylation complexes (electron transport chain complexes I-IV and ATP synthase) and the mitochondrial ribosome. To assess their suitability as LMs, we curated spatial information from the published literature and high-resolution structures (Supplementary Data 5 ), which led to the identification of another 218 LM candidates. To assess the robustness of the spatial information delivered by all LM candidates, we extracted the sub-network of these proteins and evaluated it for consistency. We deemed information as conflicting whenever the cross-links of a protein led to unreconcilable sub-compartment annotations. Among 4502 cross-links, 12 contradictory cross-linked residue pairs were found, meaning that 99.7 % of cross-links among LM candidates are internally consistent and support the known LM localizations. The 12 cross-links providing contradictory information might be caused by proteins with multiple sub-compartmental localizations or false-positive cross-link identifications. After removing LM candidates with conflicting cross-link information, 244 high-confidence LMs remained, including 31 of the 50 most connected proteins and 213 well-characterized mitochondrial proteins (Fig. 1D and Supplementary Data 5 ). Importantly, the first-tier interactors of these LMs (i.e. proteins connected through direct cross-links) cover 72% of the XL-based network, or 55% when only considering LMs that are among the 50 most abundant proteins (Fig. 1 C, E ). This confirms that XL-MS readily captures well-connected LMs that make the majority of the detected interactome amenable to CLASP localization annotations. Importantly, these annotations span all mitochondrial sub-compartments, showing that CLASP allows profiling multiple sub-compartments in parallel and determining protein localizations with sub-organelle resolution.
Having shown that the faithful capture of LMs is feasible in mitochondria, we wanted to assess whether this concept holds true when applying a different XL-MS workflow and studying another organelle. We selected synaptic vesicles (SVs) isolated from mouse brain. SVs are single membrane-bound compartments for the storage of neurotransmitters. The constitutive SV proteome is small – a previous study of rat SVs suggested that less than 50 proteins are present with at least one copy number per compartment 37 – and essential SV functions are mediated by integral or associated membrane proteins 38 , 39 . Many of these have a well-established localization/topology and thus are well suited to independently test our LM concept.
Whereas mitochondrial samples were prepared using DSSO cross-linking and cross-link enrichment by strong cation exchange chromatography, mouse brain SVs were cross-linked using the membrane-permeable, enrichable, and MS-cleavable DSBSO cross-linker 40 , and subjected to click-chemistry-based enrichment and subsequent size exclusion chromatography. We identified 5456 cross-links from mouse brain SVs by imposing a 2% FDR at the level of unique cross-linked residue pairs. The dataset includes 417 protein-protein connections (Supplementary Data 6 ). We focused on 28 proteins with established SV membrane localization/topology (Supplementary Data 7 ), qualifying as LMs according to the criteria we established above. Among these LMs, we identified 181 intra-protein and 84 inter-protein cross-links corresponding to 36 protein-protein connections. Our data showed 100% agreement with the known localization/topology of the 28 LMs (Supplementary Fig. 5 ). This confirms that XL-MS data are very likely to yield internally consistent LMs, and thus meet all criteria for performing CLASP.
CLASP facilitates efficient protein localization mapping
After validating the CLASP concept, we analyzed the CLASP predictions for DSSO-cross-linked mitochondria in more detail. We found that 91% of the CLASP annotations within our dataset are unambiguous, meaning that all LMs support the same subcellular localization. These 91% include 49% (146 proteins) confirming previous reports, 3% (9 proteins) contradicting published localization data, and 39% (115 proteins) presenting spatial and topological information for previously unannotated proteins (Fig. 2A ). CLASP suggests sub-compartment localizations for 97 hitherto unmapped proteins, revises the topology of 24 membrane proteins (see section “CLASP characterizes the topologies of membrane proteins” below), and discovers 3 mitochondria-associated proteins.
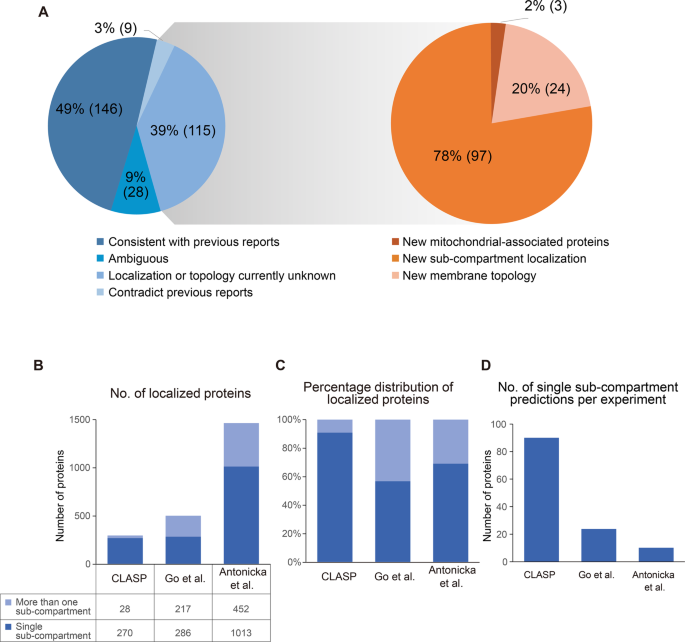
A Comparison of CLASP annotations to published protein localization information (blue pie chart, left) and breakdown of CLASP annotations that disagree with previous reports or relate to previously unannotated proteins (orange pie chart, right). Annotations of individual proteins are shown in Supplementary Data 5 . B , C Number ( B ) and percentage distribution ( C ) of mitochondrial protein localization annotations by CLASP and in published proximity biotinylation resources 7 , 29 . The columns are sub-divided to indicate the spatial specificity of the localization annotations. D Estimated information yield of a single CLASP experiment and single BioID experiments in 7 and 29 , calculated as the total number of single sub-compartment annotations divided by the total number of assays. Source data are provided as a Source Data file.
To further evaluate the scope of CLASP, we compared our dataset to two published BioID datasets generated in a similar system: the HEK293 cell map 7 and the HEK293 mitochondrial proximity interactome 29 (Fig. 2B, C ).
The HEK293 cell map determined mitochondrial localizations of 503 proteins by two data analysis algorithms (see Methods), but annotations are limited to three categories (matrix, IMM/IMS and OMM/peroxisome), indicating that the spatial resolution is too low to fully discern all sub-compartments. Our CLASP experiments provided localizations for fewer proteins (270) but resolved sub-compartment localizations in more detail (see Supplementary Data 5 , column ‘Subcellular location after CLASP annotation’). This allowed us, for example, to identify specific proteins for each individual sub-compartment and distinguish between proteins protruding into different adjacent sub-compartments, e.g. matrix-facing vs. IMS-facing IMM proteins. A similar spatial granularity was reported by Antonicka et al. who also captured the highest number of mitochondrial proteins (1465) (Fig. 2B ) 29 . However, the fraction of single sub-compartment annotations was lower in the Antonicka et al. dataset (69%) than in our CLASP dataset (91%, Fig. 2C ). Although proteins with more than one locale within mitochondria are possible, previous work suggests that less than 20% of the mitochondrial proteins may be dually localized 41 . This indicates that the high fraction of unambiguous sub-compartment annotations by CLASP is an accurate depiction of mitochondrial biology and a result of CLASP’s higher spatial specificity. In line with this, 158 proteins of our CLASP dataset were also identified by Antonicka et al. but 84 of them were ambiguously annotated. Reassuringly, of the 74 unambiguous protein annotations by both CLASP and Antonicka et al., 80% are consistent.
Furthermore, both BioID datasets are based on assays with 12 7 and 100 29 ectopically expressed baits from all mitochondrial sub-compartments, compared to three CLASP experiments in native mitochondria. This demonstrates that – by obviating exogenous fusion proteins and instead taking advantage of well-characterized mitochondrial proteins to determine protein localizations – CLASP substantially increases the yield of spatial information per experiment (Fig. 2D ).
CLASP reveals biologically relevant sub-compartment localizations
Having evaluated the fundamental features of CLASP, we next assessed its potential for biological discovery. We determined sub-compartment localizations for 209 non-membrane proteins, 97 of which were previously unassigned. Of these, 23 proteins were mapped to the IMS and 74 to the matrix (Supplementary Data 5 ).
We selected FAM136A for complementary validation. Confocal imaging of rat tissue 42 and multiple human cell lines (see https://www.proteinatlas.org/ENSG00000035141-FAM136A/subcellular#human ) has shown that endogenous FAM136A resides in mitochondria. MitoCarta3.0 places FAM136A in the IMS, based on APEX studies that found an enrichment of this protein in the IMS compared to the cytosol but did not consider other mitochondrial sub-compartments 30 Therefore, FAM136A’s sub-compartment localization has not been definitively characterized. Our CLASP analysis, which covered all mitochondrial sub-compartments, indeed suggests that FAM136A localizes to the IMS (Fig. 3A ). We verified this annotation by confocal and STED microscopy, showing that FAM136A co-localizes with the OMM marker TOMM20 and occurs within mitochondria (Fig. 3B, C ). Alkaline carbonate extraction (Fig. 3D ) and a protease protection assay (Fig. 3E ) further confirmed that FAM136A is not membrane-bound and resides in the IMS. Collectively, these data provide comprehensive evidence that FAM136A locates to the IMS in human cells.
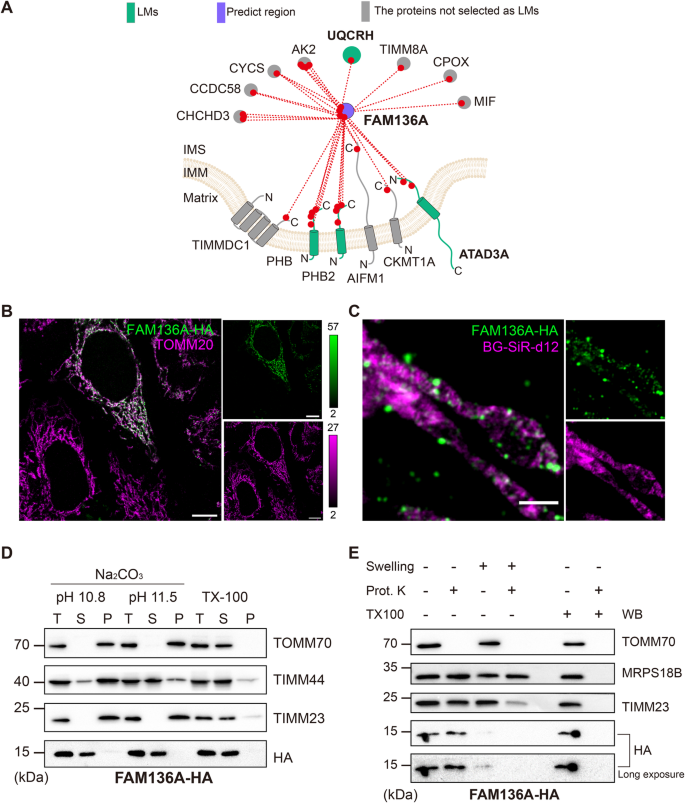
A Cross-link map of FAM136A and its interacting proteins. LMs are shown in green; FAM136A is shown in purple. The CLASP annotation of FAM136A is supported by direct connections to 1 IMS LM and 3 IMM LMs. Proteins that are not LMs but support the IMS localization of FAM136A are shown in grey. “Predict region” indicates the protein/protein region, for which a CLASP prediction was made. B Confocal fluorescence images of C-terminally HA-tagged FAM136A (FAM136A-HA) and OMM marker TOMM20 in HeLa cells (left panel). Images of untransfected cells are shown as a negative control in the bottom left. The color bar shows the contrast setting. Scale bars = 10 μm. C STED microscopy images of FAM136A-HA-transfected HeLa-COX8A-SNAP cells stained with BG-SiR-d12 (to label the IMM marker COX8A) and HA antibody. Scale bar = 1 μm. D. Alkaline carbonate extraction of mitochondria isolated from HEK293T cells overexpressing FAM136A-HA. The OMM protein TOMM70, IMM protein TIMM23 and IMM associated protein TIMM44 are used as markers for each sub-compartment. T, total mitochondrial extraction; S, supernatant; P, pellet of mitochondrial membrane. E Protease protection assay to analyze the localization of FAM136A-HA in HEK293T cells. OMM protein TOMM70, IMM protein TIMM23 and matrix protein MRPS18B are used as markers for each mitochondrial sub-compartment. Experiments in panels B-E were performed once. Source data are provided as a Source Data file.
As a second example, we followed up on NIPSNAP2/GBAS. This protein locates to the mitochondrial matrix according to MitoCarta3.0 and Abudu et al. 43 whereas Swiss-Prot (O75323, last reviewed on January 19 th , 2024) and Shanmughapriya et al. 44 annotate it as an OMM protein. According to CLASP, NIPSNAP2 resides in the matrix (Supplementary Fig. 6A ) and this is confirmed by protease protection assays (Supplementary Fig. 6B ). We additionally verified NIPSNAP2’s location by selective permeabilization experiments, in which mitochondria are treated with digitonin (permeabilizes only OMM) or triton (permeabilizes OMM and IMM). Subsequent confocal imaging reveals under which detergent conditions the proteins become accessible for the detection antibodies. OMM and IMS proteins become readily detectable upon digitonin treatment, whereas matrix proteins can only be detected in the presence of triton. These experiments show that NIPSNAP2 behaves similarly to the matrix marker SDHA and clearly different from the OMM marker TOMM20 (Supplementary Fig. 7 ). This demonstrates that NIPSNAP2 is a matrix protein and not an OMM protein in our experimental setting.
CLASP discovers mitochondria-associated proteins
We found 3 proteins, which have no previously reported association with mitochondria, cross-linked to the cytosolic side of OMM LMs (Supplementary Data 5 ). Considering the DSSO labeling radius of 4 nm, we hypothesized that these proteins may directly bind to the OMM. One of them, FAF2 (UBXD8), is known as an endoplasmic reticulum (ER) membrane protein involved in ER-associated degradation 45 . We found FAF2 directly connected to the cytosolic parts of the OMM LM VADC2 and to the ER- and mitochondria-localized protein CYB5R3 46 (Fig. 4A ), suggesting that FAF2 might localize to both mitochondrial OMM and the ER. This is supported by a protease protection assay showing a similar digestion pattern for FAF2 and the OMM protein TOMM20 (Fig. 4B ) and thus supporting the CLASP annotation of FAF2 as an OMM protein with a cytosol-facing C-terminus. Additionally, FAF2 co-localizes with TOMM20 and the ER marker Calreticulin according to confocal imaging, corroborating its localization at both organelles (Fig. 4C–E ). After we had made this discovery (published on bioRxiv in May 2022), an independent study confirmed that FAF2 (UBXD8) localizes to the ER and mitochondria in human cells 47 and a subsequent study suggested that this protein is involved in regulating mitochondria-ER contact sites 48 . These complementary findings showcase the potential of CLASP as a discovery tool that can guide functional follow-up experiments.
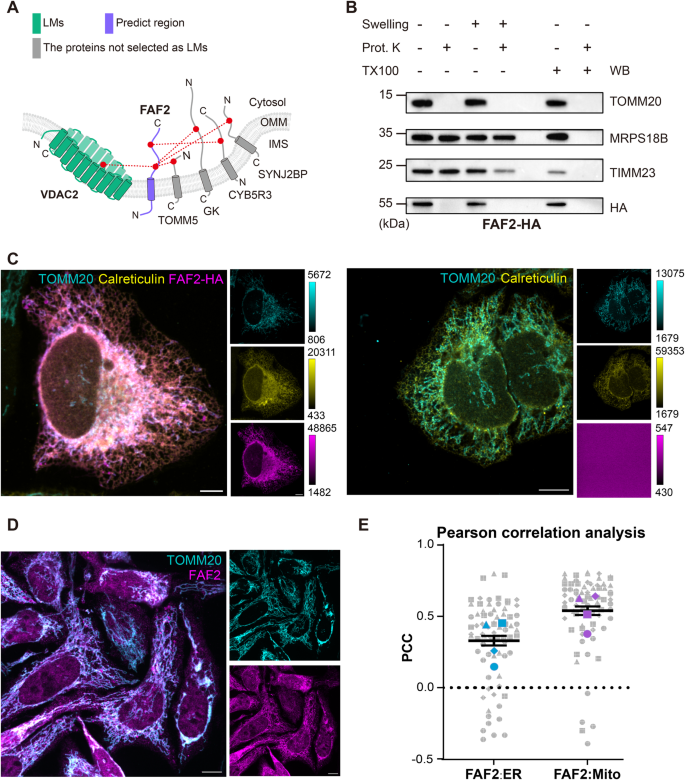
A Cross-link map of FAF2 and its interacting proteins. LMs are shown in green; FAF2 is shown in purple; proteins that are not LMs but supporting the CLASP annotation of FAF2 are shown in grey. “Predict region” indicates the protein/protein region, for which a CLASP prediction was made. B Protease protection assay to analyze the localization of HA-tagged FAF2 (FAF2-HA) in HEK293T cells. OMM protein TOMM70, IMM protein TIMM23 and matrix protein MRPS18B are used as markers for each mitochondrial sub-compartment. C Confocal fluorescence images of FAF2-HA, Calreticulin (ER marker) and TOMM20 (mitochondria marker) in HeLa cells (left panel). Untransfected negative control image for FAF2-HA staining are displayed in the right panel. Shown are representative images from a total of 4 independent experiments. The color bar shows the contrast setting. Scale bars = 10 μm. D Confocal fluorescence images of endogenous FAF2 (magenta) and TOMM20 (cyan) in HeLa cells. Scale bars = 10 μm. E Pearson correlation analysis of the co-localization of FAF2 with ER and mitochondria markers from panel C. Shown are mean values +/− SEM for n = 4 independent experiments with multiple cells per experiment. Data from each independent experiment are depicted with a distinct symbol type. Colored symbols indicate the means of the respective experiments. Experiments in panels ( B , D ) were performed once. Source data are provided as a Source Data file.
CLASP characterizes the topologies of membrane proteins
In the previous sections, we established that CLASP enables high-resolution spatial profiling owing to its clearly defined labeling radius. Another factor contributing to its high resolution is that CLASP provides sub-compartment localization information on the residue level. In other words, CLASP can distinguish regions within one protein that face different sub-compartments. This offers the opportunity to use CLASP for assessing the topology of membrane proteins. In our dataset, we annotated 58 mitochondrial membrane proteins, for which we could assign membrane regions based on previous findings and computational predictions (see Methods for details). The topological annotations of 34 proteins are consistent with previous studies. For the remaining 24 proteins, we were able to revise or complete their topological annotation based on CLASP data (Supplementary Data 5 and Fig. 5 ).
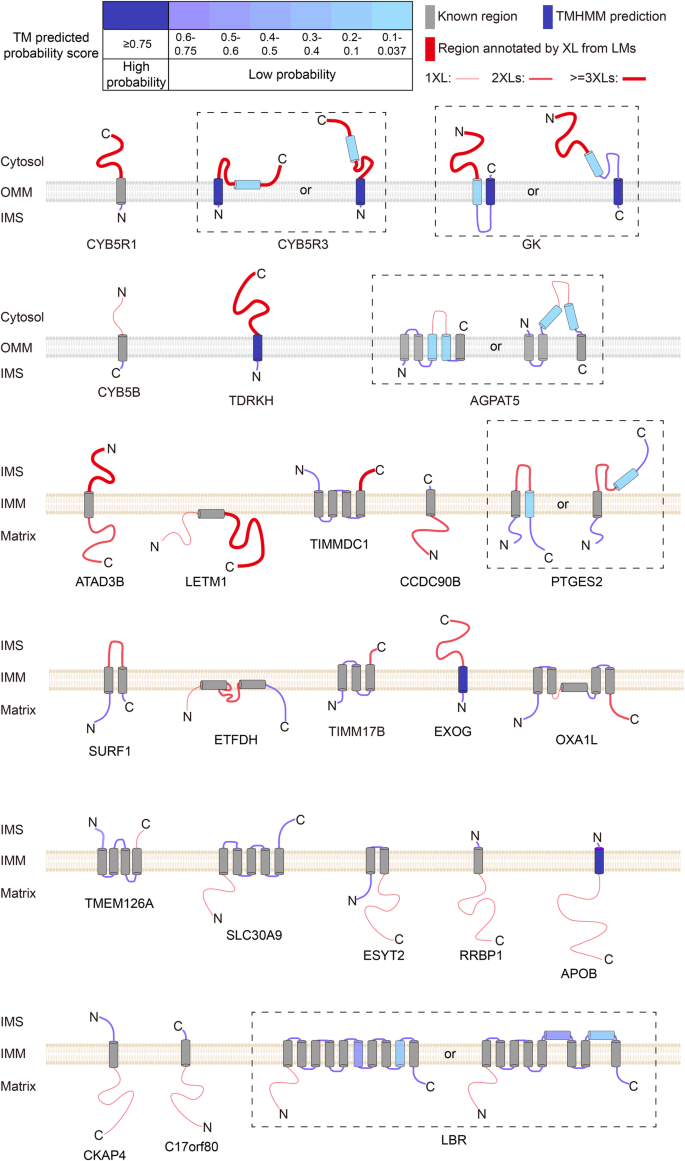
The TM regions predicted by TMHMM2.0 are gradient colored according to their posterior probabilities of TM helix; Swiss-Prot annotated TM regions are shown in grey; the regions annotated by CLASP are shown in red, the soluble regions predicted by TMHMM2.0 are shown in shades of blue. Of note, TMHMM2.0 cannot predict the localization of the soluble regions, but CLASP allows determining the orientation of the TM regions and localization of the predicted soluble regions. Dashed boxes indicate different possible topologies of one protein. Previously known and predicted TMs are included in Supplementary Data 5 .
We sought to validate two CLASP predictions: CYB5R3 as an OMM protein with a cytosol-facing C-terminus and CYB5B as an OMM protein with an IMS-facing C-terminus. Protease protection assays, confirm both of these CLASP results (Supplementary Fig. 8 ). Furthermore, we found that CLASP can help correct existing topology annotations: Swiss-Prot annotates TMEM126A as an IMM protein with matrix-facing N- and C-termini but CLASP predicts that the TMEM126A termini protrude into the IMS (Fig. 6A ). TMEM126A has a similar digestion pattern as COX8A-SNAP, which has an IMS-facing C-terminus (Fig. 6B ), again supporting the CLASP result (Fig. 6A , right panel). We additionally confirmed the IMM localization of TMEM126A through a selective permeabilization assay (Supplementary Fig. 7 ). Supporting our previous results, TMEM126A shows the highest similarity to the IMM markers TIMM23 and FAM162A. Furthermore, it shows a higher similarity to OMM marker TOMM20 than to matrix marker SDHA, confirming that its termini (the regions that are bound by the detection antibody) are facing the IMS.
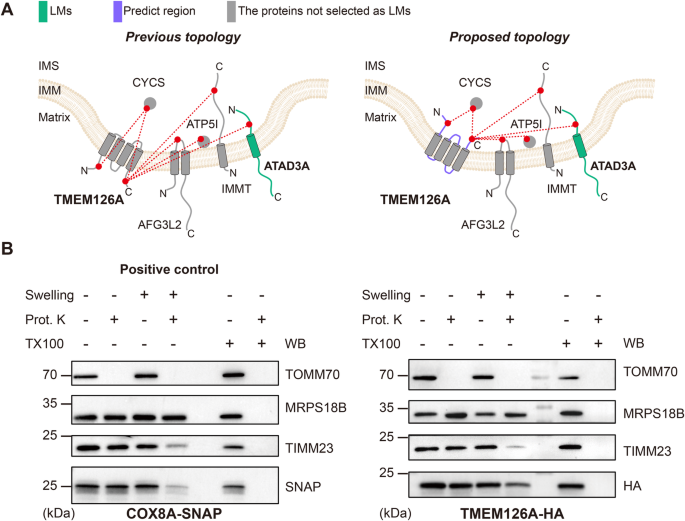
A Cross-link map of TMEM126A and its interacting proteins. Left: the cross-link map based on the previous annotation; right: the cross-link map based on the CLASP annotation. LMs are shown in green; predicted regions by TMHMM2.0 are shown in purple. IMS-localization of the TMEM126A C-terminus is supported by 1 LM. Since TMEM126A has an even number of TM regions, both termini are likely located in the same sub-compartment, which is confirmed by additional connections to proteins that are not LMs (shown in grey). “Predict region” indicates the protein/protein region, for which a CLASP prediction was made. B Protease protection assay combined with WB to analyze the localization of TMEM126A-HA in HEK293T cells. COXA8-SNAP, which has the same membrane topology as the one CLASP suggested for TMEM126A, serves as a positive control. Experiment was performed once. Source data are provided as a Source Data file.
To put these CLASP topology data in context, we compared them to a proximity biotinylation study 26 that used an APEX approach to predict IMM protein topologies in a similar HEK293 system. Lee et al. designed three APEX fusion proteins (and several more for validation experiments) to obtain membrane topology information for 60 IMM proteins, whereas CLASP provided topological insights into 42 IMM proteins from native mitochondria (Supplementary Fig. 9 ). Of the 17 protein topologies determined by both CLASP and APEX, 14 (82%) are consistent. Furthermore, 43 APEX annotations are missing in the CLASP dataset. Most of these proteins (24) have well established topologies, which is why they served as LMs in CLASP. Of the remaining APEX-only proteins, 12 were not detected by CLASP, 6 were not annotated because they did not directly cross-link to any LM, and 1 annotation was ambiguous (Supplementary Fig. 9 ). Conversely, CLASP gave topology information for 25 IMM and 16 OMM proteins not included in the APEX dataset, in addition to capturing numerous sub-compartment localizations as described above.
CLASP with an enrichable cross-linker validates and deepens spatial insights into mitochondria
Having verified several CLASP predictions through biochemical and imaging assays, we next wanted to assess whether these findings can be reproduced with a different cross-linker. Therefore, we performed CLASP on a mitochondrial XL-MS dataset generated with the MS-cleavable and enrichable DSBSO cross-linker (Supplementary Fig. 10A ). In addition, DSBSO gives us the opportunity to test whether using an enrichable cross-linker can augment CLASP.
We took the same data analysis approach as for the DSSO data: Imposing a 2% FDR (separately for inter-protein links and intra-protein links) at the level of unique cross-linked residue pairs yielded 26650 unique cross-links from 2041 proteins, including 11426 intra-protein and 15224 inter-protein cross-links corresponding to 5163 protein-protein connections (Supplementary Data 9 ). After removing proteins lacking inter-protein links or forming small disconnected clusters, we obtained a DSBSO-interactome comprising 5094 protein-protein connections (doubling the number of the DSSO interactome) among 1205 proteins (a 61% increase compared to the DSSO interactome).
For LM selection, we again focused on proteins with well-characterized sub-mitochondrial localizations, prioritizing those that are among the most connected proteins in the DSBSO interactome. The LM candidates formed 7209 cross-links amongst each other, 21 of which were contradictory to their known locations. Thus, 99.7% of cross-links among LM candidates are internally consistent and support the known LM localizations. After removing LM candidates with conflicting cross-link information, 235 high-confidence LMs remained (Supplementary Fig. 10B , Supplementary Data 10 ).
Focusing on the first-tier interactors of these LMs (i.e., proteins connected through direct cross-links), DSBSO-based CLASP yielded 420 predictions, adding or correcting the topology of 30 membrane proteins as well as the sub-mitochondrial localizations of 122 soluble proteins (Supplementary Fig. 10C , Supplementary Data 10 ). Reassuringly, DSBSO-based CLASP captured 431 out of 542 spatially annotated proteins from the original DSSO dataset (Supplementary Fig. 11 )
Mirroring our original findings with DSSO, the DSBSO-based CLASP localization predictions are highly specific with 93% of the results being unambiguous (i.e. all LMs support the same sub-compartment localization). Overall, 163 proteins were unambiguously localized by both DSSO-based and DSBSO-based CLASP (Supplementary Fig. 12 A). These overlapping predictions showed an 98% agreement (Supplementary Fig. 12B ), further validating the robustness and reproducibility of CLASP.
At the same time, the greater depth of the DSBSO dataset allowed to predict the localizations of several additional proteins. We selected four of these additional predictions (Fig. 7A ) for complementary validation by protease protection and alkaline carbonate extraction assays (Fig. 7B–E ). Specifically, we focused on
CNP (2’,3’-cyclic-nucleotide 3’-phosphodiesterase), an established mitochondrial protein known to regulate the mitochondrial permeability transition pore during cell death 49 , 50 , which has not been assigned to a sub-compartment so far and is CLASP-annotated as a matrix protein.
MIF (Macrophage migration inhibitory factor), a secretable cytoplasmic protein, which has been shown to control the regulation mitochondrial dynamics by averting apoptosis 51 . CLASP predicts that MIF resides in the IMS.
MARCKS (myristoylated alanine-rich C-kinase substrate), which was previously found to bind various cellular membranes including the OMM and be able to dissociate into the cytoplasm 52 . By contrast, CLASP localizes MARCKS to the IMS.
PFN (Profilin-1), which was recently suggested to be critical for mitochondria respiration, morphology, and dynamics, and to localize to the matrix 53 . However, CLASP predicts an IMS localization for PFN.
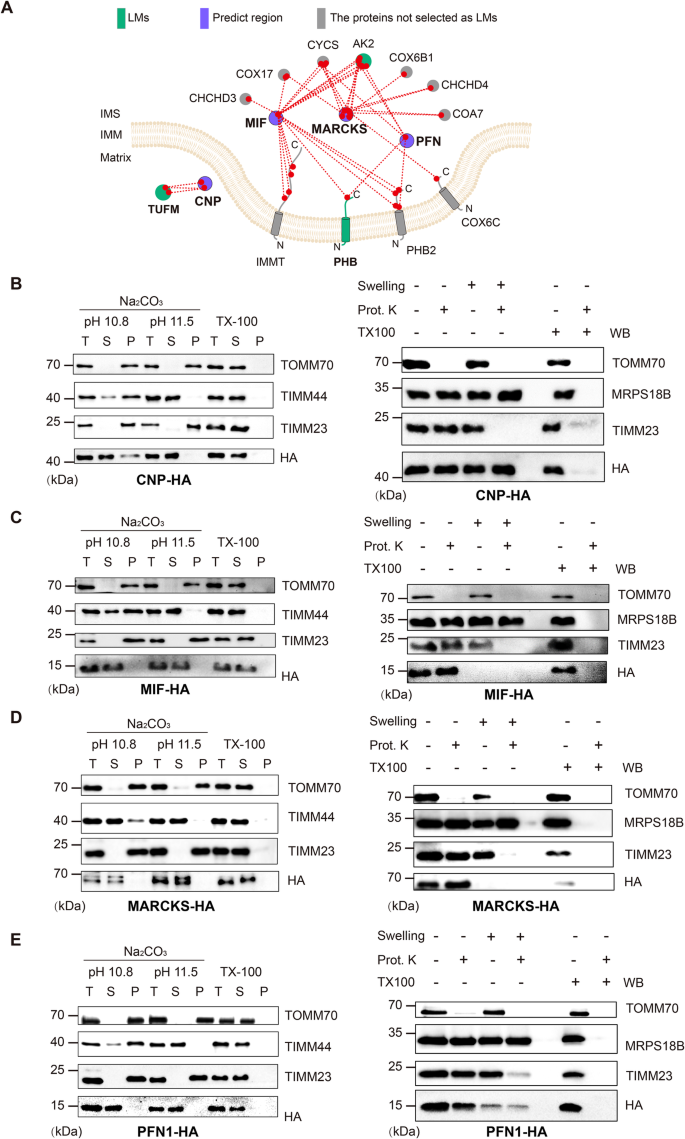
A Cross-link map of CNP, MIF, MARCKS, PFN1 and their interacting proteins based on DSBSO cross-linking. LMs are shown in green; The predicted proteins are shown in purple. Proteins that are not LMs but support the predicted localization are shown in grey. “Predict region” indicates the protein/protein region, for which a CLASP prediction was made. B – E (left panel) Alkaline carbonate extraction of mitochondria isolated from HEK293T cells overexpressing CNP-HA, MIF-HA, MARCKS-HA, PFN1-HA separately. The OMM protein TOMM70, IMM protein TIMM23 and IMM associated protein TIMM44 are used as markers for each sub-compartment. T, total mitochondrial extraction; S, supernatant; P, pellet of mitochondrial membrane. B – E (right panel) Protease protection assays to analyze the localization of CNP-HA, MIF-HA, MARCKS-HA, PFN1-HA in HEK293T cells separately. OMM protein TOMM70, IMM protein TIMM23 and matrix protein MRPS18B are used as markers for each mitochondrial sub-compartment. Experiments in ( B – E ) were performed once. Source data are provided as a Source Data file.
In all four cases, protease protection and alkaline carbonate extraction assays confirmed that the proteins are not membrane bound and reside in the sub-compartment predicted by CLASP (Fig. 7B–E ). That said, it is important to bear in mind that protein locations may change upon certain molecular and environmental triggers. For example, the localization of MARCKS has previously been shown to depend on post-translational modifications 52 Additionally, proteins may reside in more than one compartment and their dominant location may vary. This might be the case for PFN, as its band pattern in our protease protection assay (Fig. 7E ) suggests IMS as the main locale but does not fully exclude a second localization in the matrix. More generally, we cannot exclude that the localizations found here in HEK293 cells may differ from the localizations in other biological systems or conditions. Nonetheless, our biochemical data further support the accuracy of the CLASP predictions.
A Python workflow for automated CLASP predictions
Finally, we sought to increase the general accessibility of CLASP by developing a Python tool that automates LM selection and spatial predictions. The tool only requires an XL-MS data table and protein localization annotations from Swiss-Prot as input. First, it will automatically select LMs based on the Swiss-Prot annotations. Second, it will predict protein localizations and membrane topologies by combining cross-link information and defined LMs. The pipeline gives users the opportunity to manually add more LMs as this may increase the confidence of CLASP predictions. In this regard, we found that protein abundance is a useful metric to identify additional LM candidates, since more abundant proteins not only are more likely to be biologically well characterized but also tend to have a higher number of cross-linking-partners, i.e. proteins for which CLASP predictions can be made (Supplementary Fig. 13 ).
We used our DSSO dataset to compare the tool-based CLASP predictions (Supplementary Data 11 ) to our original CLASP results (Supplementary Data 5 ). Reassuringly, feeding all our manually selected LMs into the Python tool results in predictions that are 100% identical to our original DSSO-based CLASP results, confirming the functionality of our software. When relying on the tool-based automated LM selection, 98.8% of cross-links among the candidate LMs agreed with their reported locations. After removing LMs with conflicting cross-link information, we obtained 72 LMs, which yielded almost only unambiguous localization predictions (95%). The vast majority of the automatically obtained LMs and first-tier interactors have also been found using our original manual approach (298 out of 343, Supplementary Fig. 14 ). For 98% (292 proteins) of the overlapping identifications, automated and manual CLASP give the same annotation of sub-compartment localization and membrane topology. Overall, these results demonstrate that the developed Python tool offers an easy and reliable option for performing CLASP.
Over the last two decades, XL-MS has become an established tool in structural biology, complementing methods such as X-ray crystallography and cryoEM with low-resolution structural information on purified proteins and protein complexes in solution. Recent methodological advancements further expanded the scope of XL-MS to more complex biological systems 10 , 15 , but XL-MS applications remained focused on the structural analysis of proteins and discovery of protein interactions. In this study, we have developed a framework that broadens the application of XL-MS to spatial proteome profiling. Using purified human mitochondria as a model system, our CLASP approach allowed us to confirm existing spatial annotations for 146 proteins and add localization and topology data for 124 proteins (Fig. 2A and Supplementary Data 5 ). The scope of CLASP is most comparable to proximity labeling-based spatial proteomics methods, in particular APEX/BioID-derived strategies. However, a look at previous studies reveals important differences.
First, several proximity labeling-based spatial proteomics studies also took advantage of the unique morphology of mitochondria and used it as a model system for method benchmarking. These studies demonstrated, for example, the capabilities of APEX-based proximity labeling to reveal protein sub-compartment localization 27 , 30 , membrane protein topology 26 , and membrane contact sites 25 . Characterizing each of these spatial features required the design of dedicated proximity labeling experiments, whereas we have shown that CLASP can provide insights into all these aspects simultaneously in one experiment and from native mitochondria. Accordingly, CLASP substantially increases the yield of spatial information per experiment (Fig. 2D ). The main reason for this fundamental difference is that APEX/BioID methods require genetic engineering of fusion proteins targeted to a specific localization. While this strategy has undisputed advantages for profiling cell type- or localization-specific proteomes in organisms 54 , 55 , 56 , it takes substantial efforts to generate different constructs and to validate the correct localizations of the fusion proteins. Furthermore, the fusion proteins need to be ectopically expressed, which may affect the cellular status and thereby the spatial distribution of the endogenous proteins-of-interest.
Second, APEX/BioID as well as all other spatial proteomics methods derive spatial information from quantitative proteomics data and therefore must rely on statistical methods to determine which proteins are significantly enriched in one location vs another. By contrast, CLASP does not require quantitative information because it derives spatial predictions directly from qualitative XL-MS data (i.e. cross-link identifications that pass the FDR cutoff and other filtering criteria). That said, CLASP can, in principle, be applied to any comprehensive proteome-wide XL-MS dataset from an intact biological system and quantitative proteome-wide XL-MS may be used in the future to study changes of protein localizations.
A third important difference between CLASP and APEX/BioID approaches is the spatial resolution (Supplementary Fig. 15 ). The BioID resolution is limited to ca. 10 nm 12 . While there have been attempts to reduce the APEX labeling radius 57 , the free diffusion of APEX-generated radicals remains difficult to control. This may be a reason for the vast differences in estimated APEX labeling radii (ca. (20 nm 13 and 269 ± 41 nm 9 ). The comparably large labeling radii in APEX/BioID potentially compromise their utility for the highly selective labeling of specific cellular microenvironments. In support of this notion, we provide evidence that, compared to existing proximity labeling resources, CLASP yields a larger fraction of specific localization annotations (Fig. 2C ).
The localization confidence of CLASP is further increased by its ability to consider multiple LMs. This feature allows cross-validating spatial annotations through LMs with overlapping interactors and verifying the fidelity and coherence of the LMs themselves. Furthermore, we have shown that LM selection in CLASP is automatable through computational approaches. While the Python tool we developed fully relies on Swiss-Prot information that may be augmented by user input, we envision that future developments may result in more sophisticated software tools that can predict the most powerful LM combination based on the architecture of the XL-based network and existing localization evidence.
The reliance of CLASP on LMs also means that its predictive power will be lower for biological systems with fewer well-characterized LMs. However, decades of spatial biology research have generated a wealth of protein localization information, yielding hundreds of potential LMs particularly for the most relevant biological systems in fundamental and clinical research. CLASP is ideally suited to fill the remaining blank spots in the spatial protein maps of these systems because it can take advantage of existing localization data to locate previously unmapped proteins. At the same time, CLASP is still applicable to systems with sparse endogenous LMs. CLASP annotations in such systems could be improved by ectopically expressing tagged constructs as exogenous LMs, which would be conceptually more similar to the APEX/BioID fusion protein approach but still offer the benefits of CLASP’s higher spatial resolution.
Our benchmarking of CLASP omitted an analysis of its temporal resolution, which will chiefly depend on the choice of cross-linker. The DSSO and DSBSO cross-linking reactions performed in this study usually require at least 15 min incubation time during which proteins may still be partially mobile. Other cross-linking chemistries with reaction times under 30 s have been published recently 58 and may offer additional options for temporally resolved CLASP in the future. Similar developments have occurred in the proximity labeling field, where alternative labeling methods promise a better temporal control than traditional APEX/BioID approaches 59 , 60
We demonstrated the general applicability of CLASP by applying it to two sub-cellular compartments with distinct features: mitochondria and SVs. In addition, we have recently shown that the proximity information derived from XL-MS data can be used to distinguish functionally and spatially distinct protein layers within intact viral particles, which are not separated by any physical barrier 61 . Considering that CLASP is based on the same type of data and can use XL-MS datasets of any spatially defined biological system as input, these findings strongly suggest that CLASP will also be applicable to membrane-less compartments. At the same time, it is important to note that the fidelity of CLASP annotations critically depends on the comprehensiveness, accuracy, and interconnectivity of the XL-based protein network. Such detailed protein networks can be generated for purified organelles by state-of-the-art XL-MS workflows 17 , 18 , 19 , 20 , as also shown by the detection depth achieved in this study. Therefore, CLASP can readily provide detailed localization annotations for purifiable organelles, provided that sufficient input material is available. CLASP would likely be less powerful when applied to limited sample amounts (e.g. primary samples) or intact cells, since proteome coverage of most in-cell XL-MS workflows is currently still limited. However, recent technological advancements of XL-MS have brought the identification of tens of thousands of cross-links in intact cells within reach 22 , 23 . CLASP paves the way to use these data for elucidating protein localizations across the cell.
Plasmid construction
Total RNA was isolated from HEK293T cells (ATCC) by TRIzol reagent (Invitrogen) according to the manufacturer’s instruction. cDNA library was obtained by using First Strand cDNA Synthesis Kit (Thermo Fisher). For FAF2-HA and CYB5R3-HA, the open reading frames without stop codon were PCR -amplified by Phusion™ High-Fidelity DNA Polymerase (Thermo Fisher) using the forward and reverse primer pairs. The PCR product was cloned into the HindIII and BamHI sites of pcDNA3.1(+) plasmid by using GeneArt™ Seamless Cloning and Assembly Enzyme Mix (Invitrogen). OMP25-HA was cloned into the HindIII and BamHI sites of the pSNAP-N1 plasmid. pSNAPf-Cox8A Control Plasmid was a gift from New England Biolabs & Ana Egana (Addgene plasmid # 101129). The primers used for cloning were synthesized by BioTeZ Berlin-Buch GmbH and are listed in Supplementary Data 8 . All other plasmids were purchased from Absea Biotechnology Ltd. All plasmids used in this study were verified by DNA sequencing.
Cell culture and transfection
HEK293T cells (ATCC) were cultured in Dulbecco’s Modified Eagle Medium (DMEM) supplemented with 10% fetal bovine serum at 37 °C and 10% CO 2 . Plasmid transfections were performed using Lipofectamine 2000 (Invitrogen) according to the manufacturer’s instruction. Briefly, plasmid DNA and Lipofectamine 2000 were mixed with Opti-MEM separately at a ratio of 3:1 of Lipo2000 to plasmid DNA, and then Lipo2000 was added into the plasmid DNA immediately. The mixture was incubated for 20 min and added dropwise to the cell. After 48 h transfection, cells were washed and harvested with ice-cold PBS for further experiments.
Mitochondria isolation
Mitochondria isolation was modified from previous published protocols 62 as follows: Three hundred million HEK293T cells were resuspended in ice-cold buffer M (220 mM mannitol, 70 mM sucrose, 5 mM HEPES-KOH pH 7.4, 1 mM EGTA-KOH pH 7.4, supplemented with 1 mM PMSF and complete protease inhibitor EDTA-free cocktail). Cells were lysed by homogenization (25 strokes, 2 times, 900 × rpm) using dounce homogenizer. Cell debris were spun down at 800 × g for 5 min at 4 °C 2 times. The supernatants were centrifuged at 10,000 × g for 10 min at 4 °C and the pellet was collected. The pellet containing crudely purified mitochondria was further subjected to discontinuous percoll gradient centrifugation (SW41 Ti rotor, Beckman) to obtained high purity mitochondria. Protein concentration was determined by Bradford assay (Bio-rad). The crudely purified mitochondria were used for protease protection assay and alkaline carbonate extraction experiment. High purity mitochondria were used for the cross-linking experiment.
Synaptic vesicle (SV) purification
SVs were purified from C57BL/6 mouse brain as previously described 63 , 64 . The mouse work complied with all relevant ethical requirements. All animal experiments were reviewed and approved by the ethics committee of the “Landesamt für Gesundheit und Soziales” (LAGeSo) Berlin and were conducted according to the committee’s guidelines under animal experimentation permits. At the institute, the Animal Care Officer and the LAGeSo monitored compliance with all regulations. The mice were looked after by professional caretakers and checked daily. Mice were kept in an animal facility that is regularly checked for standard pathogens. Mice were kept in groups of up to six animals in standard individually ventilated cages of 524 cm 2 at (21 + /−2) °C, (50 + /−10)% relative humidity, and a 12:12 h light–dark cycle. Cages contained bedding and nesting material. Food and water were provided ad libitum.
Briefly, 10 brains from 6-week-old male and female mice were homogenized by dounce homogenizer (9 strokes, 900 × rpm) in ice-cold homogenization buffer (320 mM sucrose, 4 mM HEPES-KOH pH 7.4, supplemented with 1 mM PMSF and complete protease inhibitor EDTA-free cocktail). Cell debris were spun down at 800 × g for 10 min at 4 °C. The supernatants were centrifuged at 10,000×g for 15 min at 4 °C 2 times and the pellet (synaptosome) was collected. The pellet was osmotically lysed by ice-cold water and homogenization (3 strokes, 2000×rpm). Plasma membranes were spun down at 25,000 × g for 20 min at 4 °C (SW41 Ti rotor, Beckman). The supernatants were centrifuged at 200,000 × g for 2 h at 4 °C. The pellet (enriched SVs) was collected and used for the cross-linking experiment.
Integrity of the SVs in the preparations was assessed by negative-stain electron microscopy (Supplementary Fig. 16 ). To this end, the pellet was resuspended in homogenization buffer and diluted in phosphate-buffered saline. The sample was applied to a glow-discharged continuous carbon grid and imaged on a Zeiss 910 (FEI Morgagni).
Protease protection assay
Protease protection assays were performed following previous published protocols 65 . Briefly, freshly isolated mitochondria were suspended in SEM buffer (250 mM sucrose, 10 mM MOPS, 1 mM EDTA, pH7.2), EM buffer (10 mM MOPS, 1 mM EDTA, pH 7.2) or EM buffer containing 1% Triton X-100 respectively and incubated on ice for 25 min. Proteinase K (PK) was added into the samples and incubated for 10 min on ice. The reaction was quenched by addition of 2 mM PMSF, followed by trichloroacetic acid (TCA) precipitation. After treatment, the pellet was resuspended in SDS sample buffer and subjected to western blot analysis.
Alkaline carbonate extraction
Alkaline carbonate extraction was performed following previous published 65 . Briefly, freshly isolated mitochondria were suspended in 0.1 M Na 2 CO 3 pH 11.5, 0.1 M Na 2 CO 3 pH 10.8 or SEM buffer containing 1% Triton X-100 respectively and incubated on ice for 20 min. The samples were centrifuged at 100,000 × g for 1 h at 4 °C (S55A2 rotor, Thermo Fisher). The pellets were resuspended in SDS sample buffer and subjected to western blot analysis.
Western blot analysis
Protein samples were subjected to SDS-PAGE (14% gel) and wet-transferred to a 0.2 µm Immobilon-PSQ PVDF membrane (Millipore) at 110 V for 90 min. Blots were blocked with 5% BSA for 1 h at room temperature and incubated with corresponding primary antibodies at 4 °C overnight. The following antibodies were used in this study: anti-HA (1:1000, mouse, Abcam, ab18181); anti-SNAP (1:1000, rabbit, NEB, P9310S); anti-MRPS18B, anti-TIMM44 and TOMM70 (1:500, 1:1000, 1:500, rabbit, ProteinTech, 16139-1-AP, 13859-1-AP, 14528-1-AP); anti-TIMM23 (1:1000, mouse, DB Biotech, 611222). After washing 3 times with TBST, blots were incubated with secondary antibody peroxidase-conjugated affinipure goat anti- mouse lgG and peroxidase-conjugated affinipure goat anti-rabbit lgG (H + L, Jackson ImmunoResearch, 115-035-003, 111-035-144) for 1 h at room temperature. After washing 3 times with TBST, blots were developed with Pierce ECL Western Blotting Substrate (Thermo Fisher) and imaged by ChemiDoc MP Imager (Bio-Rad).
Cross-linking of mitochondria
The mitochondrial pellet was resuspended to 1 mg/ml in Buffer M and cross-linked by adding two aliquots of 0.5 mM disuccinimidyl sulfoxide (DSSO) or two aliquots of 0.5 mM DSBSO, each for 20 min at room temperature with constant mixing. The reaction was quenched with 20 mM Tris-HCl, pH 8.0 for 30 min at room temperature. Mitochondria were collected by centrifugation at 10000 × g for 10 min at 4 °C. DSSO cross-linking was done in three biological replicates, DSBSO cross-linking was done once.
Cross-linking of SVs
The SV pellet was resuspended to 1 mg/ml in homogenization buffer and cross-linked with 2 mM DSBSO for 15 min at room temperature with constant mixing. The reaction was quenched with 20 mM Tris-HCl, pH 8.0 for 15 min at room temperature. DSBSO cross-linking of SVs was done once. Of note, the differences in cross-linking conditions between mitochondria and SVs are a consequence of the different properties of the cross-linkers and the samples being cross-linked.
Protein digestion
Cross-linked mitochondria were digested in solution. Briefly, urea was added to the mitochondrial pellet to reach a final concentration of 8 M. Proteins were reduced and alkylated with 5 mM DTT (1 h at 37 °C) and 40 mM chloroacetamide (30 min at room temperature in the dark). Proteins were digested with Lys-C at an enzyme-to-protein ratio of 1:75 (w/w) for 4 h at 37 °C. After diluting with 50 mM TEAB to a final concentration of 2 M urea, trypsin was added at an enzyme-to-protein ratio of 1:100 (w/w) for overnight at 37 °C. The digestion was quenched by adding formic acid to a final concentration of 1%. Peptides were desalted with Sep-Pak C18 cartridges (Waters) according to the manufacturer’s protocol, dried in a vacuum concentrator.
Cross-linked SVs were precipitated by chloroform-methanol method. Briefly, three sample volumes of water, four sample volumes of methanol and one sample volume of chloroform were added into the sample and mixed vigorously. The sample was centrifuged at 15,000 × g for 10 min at room temperature using a tabletop centrifuge, then the upper layer was carefully removed. Three sample volumes of methanol were added and the sample was centrifuged at 13,000 × g for 10 min. The precipitated cross-linked SV proteins in the pellet were then digested using the same method as described above.
Enrichment of DSBSO-cross-linked mitochondria and SV peptides
The digested cross-linked mitochondria and SV peptides were enriched by dibenzocyclooctyne (DBCO)-coupled sepharose beads. Briefly, the mitochondrial or SV peptides were resuspended to 3 mg/ml in PBS and were then added to the prewashed DBCO beads for incubation overnight at room temperature with constant mixing. The bead-to-peptide ratio was 20 µl beads (40 µl slurry) per 0.6 mg peptide. After incubation, the beads were washed with water, followed by a washing step with 0.5% SDS at 37 °C for 15 min. Next, the beads were washed thrice with 0.5% SDS, thrice with 8 M urea in 50 mM TEAB, thrice with 10% ACN and twice with water using ten bead volumes each. The cross-linked peptides were eluted with two bead volumes 10 % (v/v) trifluoracetic acid (TFA) for 2 h at 25 °C and then dried using a vacuum concentrator.
Size exclusion chromatography (SEC) fractionation of DSBSO-cross-linked mitochondrial and SV peptides
SEC fractionation was performed on 160 µg peptides using a SuperdexTM 30 Increase 3.2/300 column (GE Healthcare) on an Agilent 1260 Infinity II system. For mitochondria, a 60 min gradient was applied and 24 fractions were collected and dried in a vacuum concentrator. For SVs, a 90 min gradient was applied and 13 fractions were collected and dried in a vacuum concentrator.
Strong cation exchange (SCX) fractionation of DSSO-cross-linked mitochondrial peptides
SCX fractionation was performed on mitochondrial digested peptides using PolySULFOETHYL-ATM column (100 × 4.6 mm, 3 μm particles, PolyLC INC.) on an Agilent 1260 Infinity II UPLC system. A 95 min gradient was applied and fractions were collected every 30 sec. 50 late SCX fractions were desalted by C18 stageTip and dried in a vacuum concentrator.
High pH (HPH) fractionation of DSBSO-cross-linked mitochondrial peptides
HPH fractionation was performed on mitochondrial digested peptides after SEC fractionation using Phenomenex Gemini C18 column on an Agilent 1260 Infinity II UPLC system. A 85 min gradient was applied and 42 fractions were collected and dried under SpeedVac.
LC-MS analysis
For the analysis of DSSO-cross-linked mitochondrial peptides, collected SCX fractions were analyzed by LC-MS using an UltiMate 3000 RSLC nano LC system coupled on-line to an Orbitrap Fusion Lumos mass spectrometer (Thermo Fisher Scientific). Reversed phase separation was performed with an in-house packed C18 analytical column (Poroshell 120 EC-C18, 2.7 µm, Agilent Technologies). 3 h LC-MS runs were performed for cross-linking acquisition and 2 h runs were done for proteomic analysis. Cross-link acquisition was performed using a CID-MS2-MS3 acquisition method. MS1 and MS2 scans were acquired in the Orbitrap mass analyzer and MS3 scans were acquired in the ion trap mass analyzer. Notably, MS3 acquisitions were only triggered when peak doublets with a specific mass difference (Δ = 31.9721 Da) were detected in the CID -MS2 spectra, as this is indicative for the presence of DSSO cross-linked peptides. The following MS parameters were applied: MS resolution 120,000; MS2 resolution 60,000; charge state 4-8 enable for MS2; MS2 isolation window, 1.6 m/z; MS3 isolation window, 2.5 m/z; MS2- CID normalized collision energy, 25%; and MS3-CID normalized collision energy, 35%. For mitochondrial proteome analysis, mass analysis was performed using an Orbitrap Fusion mass spectrometer (Thermo Fisher Scientific) with a HCD-MS2 acquisition method. MS1 scans were acquired in the Orbitrap mass analyzer and MS2 scans were acquired in the ion trap mass analyzer. The following MS parameters were applied: MS resolution 120,000; MS2 resolution 60,000; charge state 2-4 enable for MS2; MS2 isolation window, 1.6 m/z; MS2- HCD normalized collision energy, 30%.
For the analysis of DSBSO-cross-linked samples, collected SEC fractions (SV peptides) or HPH fractions (mitochondrial peptides) were analyzed by LC-MS using an UltiMate 3000 RSLC nano LC system coupled on-line to an Orbitrap Fusion Lumos mass spectrometer with a FAIMS Pro device (Thermo Fisher Scientific). Reversed phase separation was performed with an in-house packed C18 analytical column (Poroshell 120 EC-C18, 2.7 µm, Agilent Technologies). 3 h LC-MS runs were performed for cross-linking acquisition. Cross-link acquisition was performed using a stepped higher collisional dissociation (stepHCD) MS2 acquisition method. MS1 and MS2 scans were acquired in the Orbitrap mass analyzer. The following MS parameters were applied: MS resolution 120,000; MS2 resolution 60,000; charge state 4-8 enable for MS2; MS2 isolation window, 1.6 m/z; stepped normalized collision energy, 19-25-30% with FAIMS voltages set to −50, −60, and −75.
MS data analysis
For cross-linking samples, raw data were converted into MGF files in Proteome Discoverer (version 2.4). Data analysis was performed using a stand-alone version of XlinkX 66 with the following parameters: minimum peptide length 6; maximal peptide length 35; missed cleavages 3; fix modification: Cys carbamidomethyl; variable modification: Met oxidation; DSSO cross-linker =158.0038 Da (short arm = 54.0106 Da, long arm = 85.9824 Da); precursor mass tolerance 10 ppm; fragment mass tolerance 20 ppm. MS2 spectra were searched against a reduced target-decoy Swiss-Prot human database derived from proteins combining MitoCarta2.0 database and protein identification from a mitochondrial proteomic measurement. Results were reported at 2% FDR at the level of unique cross-linked residue pairs. Protein- protein interaction (PPI) network was constructed by Cytoscape software (version 3.8.2). For synaptic vesicles sample, XlinkX stand-alone with the following parameters: minimum peptide length 6; maximal peptide length 35; missed cleavages 3; fix modification: Cys carbamidomethyl; variable modification: Met oxidation; DSBSO cross-linker =308.0038 Da (short arm = 54.0106 Da, long arm = 236.0177 Da); precursor mass tolerance 10 ppm; fragment mass tolerance 20 ppm. MS2 spectra were searched against a reduced target-decoy Swiss-Prot mouse database. Results were reported at 1% FDR at CSM level. Protein- protein interaction (PPI) network was constructed by Cytoscape software (version 3.8.2).Proteomics data were analyzed using MaxQuant software (version 1.6.2.6a) with the following searching parameters: precursor mass tolerance 20 ppm, fragment mass tolerance 20 ppm; fixed modification: Cys carbamidomethylation; variable modification: Met oxidation, protein N-term Acetyl; enzymatic digestion: trypsin/P; maximum missed cleavages: 2. Database search was performed using Swiss-Prot database of all human proteins without isoform (retrieved on May 2020, containing 20,365 target sequences); false discovery rate: 1%. Intensity-based absolute quantification (iBAQ) was enabled to determine to relative abundances of proteins.
Structural validation for cross-links
Cross-links were mapped onto selected high-resolution structures from the Protein Data Bank (PDB) using Pymol 2.1.0 (Schrodinger LLC). PDB accession codes are provided in the Data Availability section. If homologous non-human structures were used, sequences were aligned to the human protein sequence using NCBI BLAST and cross-links were mapped to the aligned residues.
Confocal immunofluorescence and STED microscopy
For standard IF, HeLa cells (ATCC) and HeLa-COX8A-SNAP cells (reported in ref. 67 and kindly donated by the authors) were seeded on µ-Slide 8 Well Glass Bottom (ibidi, REF 80827) one day before transfection, cultivated in DMEM containing 10 % FBS, 1 % P/S and 1% L-Glut. After 24 h, the transfected cells were fixed with PBS containing 4% paraformaldehyde (PFA) and 4% sucrose for 10 min at room temperature. Afterwards, fixation was quenched by removing PFA and adding PBS containing 0.1 M glycine and 0.1 M NH 4 Cl for 10 min at room temperature. Cells were permeabilized by PBS containing 0.15 % TritonX-100 for 10 min at room temperature and washed by PBS two times. Then sequentially incubated in blocking buffer (PBS containing 1% BSA and 6% NGS) for 30 min at room temperature, blocking buffer containing primary antibodies for 1 h at room temperature. After three washes in blocking buffer, the cells were incubated in blocking buffer containing secondary antibody for 30 min at room temperature. After three washes in blocking buffer, the cells were imaged using confocal microscopy.
For STED microscopy using HeLa-COX8A-SNAP, cells were incubated for 1 h with 1 µM BG-SiR d12, followed by three times washing with DMEM medium shortly before fixation. Cells were fixated in culture, by extraction of half of the DMEM medium and adding PBS containing 4% paraformaldehyde (PFA) and 4% sucrose for 20 min at 37 °C. Afterwards, the standard IF protocol was followed.
For selective permeabilization experiments, cells were seeded onto µ-Slide 8 Well Glass Bottom (ibidi, REF 80827). Transient transfection with selected candidate plasmids was performed the next day. After standard fixation with 4% PFA the following day, samples were subjected to two rounds of IF staining. In the first round, cells were permeabilized with 0.02% digitonin in PBS for 10 min before blocking according to the standard protocol. Following an initial incubation with the specific primary antibody, samples were incubated with species-specific secondary antibodies coupled to a cyanine-based fluorescent dye with 568 nm excitation (CF®568, Biotum). After extensive washing, cells were further permeabilized with 0.15% TritonX100 in PBS for 10 min, followed by blocking. To visualize the newly exposed epitopes, the samples were again incubated with the specific primary antibodies. This time a secondary antibody with 640 nm excitation was used (CF®640 R, Biotum).
Confocal images for the standard IF were taken on a Nikon spinning disc microscope (Yokogawa spinning disk CSU-X1) equipped with the following lasers (488 nm, 561 nm and 638 nm), a 60 × oil objective (Plan-Apo, NA 1.40 Nikon), a 40 × air objective (Plan Apo, NA 0.95), an Andor camera (AU888, 13 μm/pixel) and NIS-Elements software (Nikon).
Confocal images for the selective permeabilization experiments were taken on a Nikon spinning disc microscope (Yokogawa spinning disk CSU-W1 with 50um pinholes) equipped with the following lasers (488 nm, 561 nm and 638 nm), a 60 × oil objective (Plan-Apo LambdaD, NA 1.42 Nikon), a 40 × air objective (Plan Apo, NA 0.95), an sCMOS camera (pco.edge bi4.2, 6.5 μm/pixel) and NIS-Elements software (Nikon).
STED images were taken on a STEDYCON system (Abberior Instruments) mounted onto a Nikon TI Eclipse with a nanoZ Controller (Prior) and controlled by Micro-Manager ( https://micro-manager.org/ ). Imaging was done with two different pulse Diode lasers (561 nm, 640 nm) for excitation paired with two single counting avalanche photodiodes (650−700 nm, 575–625 nm) for detection, a 775 nm STED laser for depletion and an optic tunable filter to modulate all laser beams for confocal imaging. Images were captured by using an 100 × 1.45 NA lambda oil objective lens with a fixed pixel size set to 20 nm for STED images.
The following primary antibodies were used in FAF2 experiment: anti-mouse-TOMM20 (1:100, Santa Cruz, sc-17764, RRID:AB 628381), anti-rat-HA (1:100, Chromotek, 7c9-100, RRID:AB_2631399), anti-rabbit-Calreticulin (1:100, Thermo, PA3-900, RRID:AB_325990), anti-rabbit-FAF2 (Proteintech, 16251-1-AP, RRID:AB_2262469) The following primary antibodies were used in FAM136A experiment: anti-mouse-TOMM20 (1:100, Santa Cruz, sc-17764, RRID:AB_628381), anti-rabbit-HA (1:100, Cayman, cay162200-1, RRID:AB_327903). The following primary antibodies were used in selective permeabilization experiment: anti-mouse-TOMM20 (1:100, Santa Cruz, sc-17764, RRID:AB_628381), anti-mouse-HA (1:100, Santa Cruz, sc-7392, RRID:AB_627809), anti-mouse-TIMM23 (1:50, BD Biosciences, 611222, RRID:AB_398754), anti-rabbit-COX4 (1:100, Cell Signaling, 4850, RRID:AB 2085424), anti-mouse-SDHA (1:50, Abcam, ab14715, RRID:AB 301433)
The following secondary antibodies were used in FAF2 and FAM136A experiment: anti-rat-AF488 (1:200, Invitrogen, A11006, RRID:AB_2534074), anti-mouse-AF594 (1:200, Thermo, A11032, RRID:AB_2534091) and anti-rabbit-AF647 (1:200, Thermo, A21244, RRID:AB_2535812). The following secondary antibodies were used in selective permeabilization experiment: anti-mouse- CF®568 (Biotum, 20105, RRID:AB 10557030), anti-rabbit-CF®568 (Biotum, 20098, RRID:AB 10557118), anti-mouse-CF®640 R (Biotum, 20177, RRID:AB 10853623), anti-rabbit-CF®640 R (Biotum, 20178, RRID:AB 10852688).
Imaging analysis
Images were processed using FIJI analysis software ( https://imagej.net/software/fiji/ ). Co-localization was analyzed using the Coloc2 plugin on two-color confocal images ( https://imagej.net/plugins/coloc-2 ). Bisection threshold regression was applied to multiple manually selected ROIs per overview image with PSF 3 to determine co-localization between both acquired channels. The Pearson’s R value above the threshold was reported as the co-localization metric, unless otherwise stated. For the FAF2 co-localization analysis, a dataset consisting of two conditions over four experiments with a total of 150 images was used. Co-localization was determined between the FAF2 signal and the respective organelle of interest, either mitochondria (TOMM20) or ER (Calreticulin). For the selective permeabilization assays, a dataset from 3 separate experiments with 30 images per candidate was used. Co-localization was determined between the two different permeabilization conditions per candidate.
Computational predictions for TM regions
For the 298 first-tier interactors of the 244 LMs, we used the software TMHMM 68 to predict the transmembrane regions. Here we used the posterior probability to show the prediction confidence. 53 transmembrane proteins with known topology in 244 LMs were used to define the probability score range. Posterior probability score above 0.75 was defined as high confidence transmembrane region, 0.037–0.75 as potential transmembrane region, below 0.037 as soluble region.
Comparison of the DSSO-cross-linking-based mitochondrial proteome to published mitochondrial proteome resources
We performed a comparative analysis on mitochondria level without considering sub-compartments. The following datasets were included:
-Our CLASP analysis of DSSO-cross-linked mitochondria, which included 748 proteins that can be confidently assigned to this organelle (albeit not always with sub-compartment information)
-The DOMs dataset from Schessner et al. (Supp. Data 1 in ref. 35 ), which reported 958 mitochondrial assignments
-The LOPIT-DC and HyperLOPIT datasets from Geladaki et al. (Supp. Data 1 and 2 in 36 ), which assigned 464 and 548 mitochondrial proteins, respectively, in U-2 OS cells
-The MitoCoP database from Morgenstern et al. (Table S1 in ref. 34 ), which – after removal of duplicate entries - contains 1137 mitochondrial and mitochondrial-associated proteins
-The MitoCarta3.0 database described by Rath et al. 33 (available at https://www.broadinstitute.org/files/shared/metabolism/mitocarta/human.mitocarta3.0.html ), comprising 1136 human proteins.
Comparison of CLASP data to published proximity biotinylation datasets
We re-analyzed data from the HEK293 cell map 7 , the HEK293 mitochondrial proximity interactome 29 and the IMM protein architecture map 26 .
Our analysis of the Go et al. dataset was based on Supplementary Table 9 of the original paper 7 , which shows cellular sub-compartment predictions for the confidently identified proximity interactors (filtered at 1% Bayesian FDR) based on two prediction algorithms – Non-negative Matrix Factorization (NMF) and Spatial Analysis of Functional Enrichment (SAFE). We filtered for proteins for which both algorithms suggested a mitochondrial localization. The number of predicted sub-compartments was determined using NMF predictions since SAFE did not provide any single sub-compartment predictions.
For the Antonicka et al. dataset, we determined the number of confidently predicted proteins by filtering their full proximity interactome (Table S4 in ref. 29 ) at a Bayesian false discovery rate threshold of 1% (i.e. the same cutoff as in the original publication). We then used the reported sub-compartment annotations of each BioID bait (Fig. 2B in ref. 29 ) to determine in which sub-compartments the reported prey proteins were detected.
For the comparison shown in Fig. 2B–D , proteins predicted to reside in a single sub-compartment were classified in “single sub-compartment” group. Proteins with ambiguous localization or predicted to reside in more than one sub-compartment were classified in “more than one sub-compartments” group (see Supplementary Data 5, column “single sub-compartment localization (Yes or No)”).
For the Lee et al. dataset, we considered all transmembrane IMM proteins, for which the authors proposed or confirmed topological information (reported in Data Set S7 of 26 ).
CLASP Python tool
The developed python pipeline is divided in two parts. Users need to load an XL-MS data table and a TSV file downloaded from Swiss-prot/Uniprot with localization and topology annotations. The first part of the pipeline will automatically select LMs from proteins with unequivocal localization annotation in the Swiss-Prot database. It also offers the possibility to add and modify LMs and their annotations. The second part of the pipeline will predict protein locations and membrane protein topologies based on the provided cross-link information and the LMs defined in the first part. The resulting CLASP annotations will be automatically saved in an output table. Full instructions how to execute this tool are provided on GitHub (see Code Availability statement below).
Reporting summary
Further information on research design is available in the Nature Portfolio Reporting Summary linked to this article.
Data availability
All CLASP predictions and LMs are reported in Supplementary Data 5 (DSSO-cross-linked mitochondria, manual CLASP), Supplementary Data 7 (SVs), Supplementary Data 10 (DSBSO-cross-linked mitochondria), and Supplementary Data 11 (DSSO-cross-linked mitochondria, automated CLASP). The underlying cross-link identifications are reported in Supplementary Data 1 – 4 (DSSO-cross-linked mitochondria), Supplementary Data 6 (SVs), and Supplementary Data 9 (DSBSO-cross-linked mitochondria). The mass spectrometry raw data have been deposited to the PRIDE repository with the dataset identifiers PXD032132 and PXD046382 .
For validating the mitochondrial localization of endogenous Fam136A in human cells, we used publicly available data from Human Protein Atlas ( https://www.proteinatlas.org/ENSG00000035141-FAM136A/subcellular#human ).
For comparing CLASP to published spatial proteomics studies, we used:
• The Go et al. dataset (Supp. Table 9 of the original paper 7 )
• The Antonicka et al. dataset (Table S4 in 29 )
• The Lee et al. dataset (Data Set S7 of 26 )
• The DOMs dataset from Schessner et al. (Supp. Data 1 in 35 )
• The LOPIT-DC and HyperLOPIT datasets from Geladaki et al. (Supp. Data 1 and 2 in 36 )
• The MitoCoP database from Morgenstern et al. (Table S1 in 34 )
• The MitoCarta3.0 database described by ref. 33 (available at https://www.broadinstitute.org/files/shared/metabolism/mitocarta/human.mitocarta3.0.html )
For confirming the labeling radius of DSSO-based CLASP, the following publicly available PDB structures were used:
• The mitochondrial electron transport chain complexes I, II, III and IV with PDB accession codes 5XTD , 1ZOY , 5XTE and 5Z62 , respectively
• TOMM complex with PDB accession code 7CK6
• TIMM22 complex with PDB accession code 7CGP
• TIMM9-TIMM10 complex with PDB accession code 2BSK
• Succinyl-CoA ligase complex SUCLG1-SUCLG2 with PDB accession code 6G4Q
• MCAD-ETF complex with PDB accession code 1T9G
• 39S mitoribosome with PDB accession code 7OIE
• Frataxin bound iron sulfur cluster assembly complex with PDB accession code 6NZU
• Iron sulfur cluster assembly with PDB accession code 5KZ5
• Calcium uniporter homocomplex with PDB accession code 6WDN
• Transcription ignition complex with PDB accession code 6ERQ
• Mitochondrial DNA replicase with PDB accession code 4ZTU
• Trifunction protein with PDB accession code 6DV2
• FIS with PDB accession code 1PC2
• CYB5R3 with PDB accession code 1UMK
• TIMM44 with PDB accession code 2CW9
• MUT with PDB accession code 3BIC
• VDAC1 with PDB accession code 6G6U
• VDAC2 with PDB accession code 4BUM
• SLC25A13 with PDB accession code 4P5W
• COQ8A with PDB accession code 4PED
• EXOG with PDB accession code 5T5C
• CLPP with PDB accession code 6DL7
• PHB2 with PDB accession code 6IQE
• OPA1 with PDB accession code 6JTG
• TRAP1 with PDB accession code 4Z1L
• CKMT1A with PDB accession code 1QK1
• PNPase with PDB accession code 5FZ6
A source data file is provided with this manuscript. Source data are provided with this paper.
Code availability
The Python tool for automated CLASP, a user guide, and example input and output data are provided through Github ( https://github.com/theliulab/protein_location_prediction , available under a MIT license) and Zenodo ( https://doi.org/10.5281/zenodo.10824759 , available under a Creative Commons Attribution 4.0 International license).
Lundberg, E. & Borner, G. H. H. Spatial proteomics: a powerful discovery tool for cell biology. Nat. Rev. Mol. Cell Biol. 20 , 285–302 (2019).
Article CAS PubMed Google Scholar
Christopher, J. A. et al. Subcellular proteomics. Nat. Rev. Methods Prim. 1 , 32 (2021).
Article CAS Google Scholar
Borner, G. H. H. Organellar Maps Through Proteomic Profiling - A Conceptual Guide. Mol. Cell Proteom. 19 , 1076–1087 (2020).
Article Google Scholar
Christoforou, A. et al. A draft map of the mouse pluripotent stem cell spatial proteome. Nat. Commun. 7 , 8992 (2016).
Article PubMed Google Scholar
Krahmer, N. et al. Organellar Proteomics and Phospho-Proteomics Reveal Subcellular Reorganization in Diet-Induced Hepatic Steatosis. Dev. Cell 47 , 205–221 e207 (2018).
Orre, L. M. et al. SubCellBarCode: Proteome-wide Mapping of Protein Localization and Relocalization. Mol. Cell 73 , 166–182 e167 (2019).
Go, C. D. et al. A proximity-dependent biotinylation map of a human cell. Nature 595 , 120–124 (2021).
Article ADS CAS PubMed Google Scholar
Choi, C. R. & Rhee, H. W. Proximity labeling: an enzymatic tool for spatial biology. Trends Biotechnol. 40 , 145–148 (2021).
Oakley, J. V. et al. Radius measurement via super-resolution microscopy enables the development of a variable radii proximity labeling platform. Proc. Natl Acad. Sci. USA 119 , e2203027119 (2022).
Article CAS PubMed PubMed Central Google Scholar
O’Reilly, F. J. & Rappsilber, J. Cross-linking mass spectrometry: methods and applications in structural, molecular and systems biology. Nat. Struct. Mol. Biol. 25 , 1000–1008 (2018).
Merkley, E. D. et al. Distance restraints from crosslinking mass spectrometry: mining a molecular dynamics simulation database to evaluate lysine-lysine distances. Protein Sci. 23 , 747–759 (2014).
Kim, D. I. et al. Probing nuclear pore complex architecture with proximity-dependent biotinylation. Proc. Natl Acad. Sci. USA 111 , E2453–E2461 (2014).
Martell, J. D. et al. Engineered ascorbate peroxidase as a genetically encoded reporter for electron microscopy. Nat. Biotechnol. 30 , 1143–1148 (2012).
Geri, J. B. et al. Microenvironment mapping via Dexter energy transfer on immune cells. Science 367 , 1091–1097 (2020).
Article ADS CAS PubMed PubMed Central Google Scholar
Liu, F. & Heck, A. J. Interrogating the architecture of protein assemblies and protein interaction networks by cross-linking mass spectrometry. Curr. Opin. Struct. Biol. 35 , 100–108 (2015).
Mendes, M. L. et al. An integrated workflow for crosslinking mass spectrometry. Mol. Syst. Biol. 15 , e8994 (2019).
Fasci, D., van Ingen, H., Scheltema, R. A. & Heck, A. J. R. Histone Interaction Landscapes Visualized by Crosslinking Mass Spectrometry in Intact Cell Nuclei. Mol. Cell Proteom. 17 , 2018–2033 (2018).
Gonzalez-Lozano, M. A. et al. Stitching the synapse: Cross-linking mass spectrometry into resolving synaptic protein interactions. Sci. Adv. 6 , eaax5783 (2020).
Liu, F., Lossl, P., Rabbitts, B. M., Balaban, R. S. & Heck, A. J. R. The interactome of intact mitochondria by cross-linking mass spectrometry provides evidence for coexisting respiratory supercomplexes. Mol. Cell Proteom. 17 , 216–232 (2018).
Wittig, S. et al. Cross-linking mass spectrometry uncovers protein interactions and functional assemblies in synaptic vesicle membranes. Nat. Commun. 12 , 858 (2021).
Schweppe, D. K. et al. Mitochondrial protein interactome elucidated by chemical cross-linking mass spectrometry. Proc. Natl Acad. Sci. USA 114 , 1732–1737 (2017).
Wheat, A. et al. Protein interaction landscapes revealed by advanced in vivo cross-linking-mass spectrometry. Proc. Natl Acad. Sci. USA 118 , e2023360118 (2021).
Jiang, P. L. et al. A Membrane-Permeable and Immobilized Metal Affinity Chromatography (IMAC) Enrichable Cross-Linking Reagent to Advance In Vivo Cross-Linking Mass Spectrometry. Angew. Chem. Int Ed. Engl. 61 , e202113937 (2021).
Kao, A. et al. Development of a Novel Cross-Linking Strategy for Fast and Accurate Identification of Cross-Linked Peptides of Protein Complexes. Mol. Cell. Proteom. 10 , M110.002212 (2011).
Kwak, C. et al. Contact-ID, a tool for profiling organelle contact sites, reveals regulatory proteins of mitochondrial-associated membrane formation. Proc. Natl Acad. Sci. USA 117 , 12109–12120 (2020).
Lee, S. Y. et al. Architecture Mapping of the Inner Mitochondrial Membrane Proteome by Chemical Tools in Live Cells. J. Am. Chem. Soc. 139 , 3651–3662 (2017).
Rhee, H. W. et al. Proteomic mapping of mitochondria in living cells via spatially restricted enzymatic tagging. Science 339 , 1328–1331 (2013).
Frey, T. G. & Mannella, C. A. The internal structure of mitochondria. Trends Biochem Sci. 25 , 319–324 (2000).
Antonicka, H. et al. A High-Density Human Mitochondrial Proximity Interaction Network. Cell Metab. 32 , 479–497 e479 (2020).
Hung, V. et al. Proteomic mapping of the human mitochondrial intermembrane space in live cells via ratiometric APEX tagging. Mol. Cell 55 , 332–341 (2014).
Kaake, R. M. et al. A New in Vivo Cross-Linking Mass Spectrometry Platform to Define Protein–Protein Interactions in Living Cells. Mol. Cell. Proteom. 13 , 3533 (2014).
Perkins, G. et al. Electron tomography of neuronal mitochondria: three-dimensional structure and organization of cristae and membrane contacts. J. Struct. Biol. 119 , 260–272 (1997).
Rath, S. et al. MitoCarta3.0: an updated mitochondrial proteome now with sub-organelle localization and pathway annotations. Nucleic Acids Res. 49 , D1541–D1547 (2021).
Morgenstern, M. et al. Quantitative high-confidence human mitochondrial proteome and its dynamics in cellular context. Cell Metab. 33 , 2464–2483 e2418 (2021).
Schessner, J. P., Albrecht, V., Davies, A. K., Sinitcyn, P. & Borner, G. H. H. Deep and fast label-free Dynamic Organellar Mapping. Nat. Commun. 14 , 5252 (2023).
Geladaki, A. et al. Combining LOPIT with differential ultracentrifugation for high-resolution spatial proteomics. Nat. Commun. 10 , 331 (2019).
Article ADS PubMed PubMed Central Google Scholar
Taoufiq, Z. et al. Hidden proteome of synaptic vesicles in the mammalian brain. Proc. Natl Acad. Sci. USA 117 , 33586–33596 (2020).
Li, L. & Chin, L. S. The molecular machinery of synaptic vesicle exocytosis. Cell Mol. Life Sci. 60 , 942–960 (2003).
Sudhof, T. C. The synaptic vesicle cycle. Annu Rev. Neurosci. 27 , 509–547 (2004).
Kaake, R. M. et al. A new in vivo cross-linking mass spectrometry platform to define protein-protein interactions in living cells. Mol. Cell Proteom. 13 , 3533–3543 (2014).
Vogtle, F. N. et al. Landscape of submitochondrial protein distribution. Nat. Commun. 8 , 290 (2017).
Requena, T. et al. Identification of two novel mutations in FAM136A and DTNA genes in autosomal-dominant familial Meniere’s disease. Hum. Mol. Genet 24 , 1119–1126 (2015).
Princely Abudu, Y. et al. NIPSNAP1 and NIPSNAP2 Act as “Eat Me” Signals for Mitophagy. Dev. Cell 49 , 509–525 e512 (2019).
Shanmughapriya, S. et al. SPG7 Is an Essential and Conserved Component of the Mitochondrial Permeability Transition Pore. Mol. Cell 60 , 47–62 (2015).
Mueller, B., Klemm, E. J., Spooner, E., Claessen, J. H. & Ploegh, H. L. SEL1L nucleates a protein complex required for dislocation of misfolded glycoproteins. Proc. Natl. Acad. Sci. USA 105 , 12325–12330 (2008).
Neve, E. P. A. et al. Amidoxime Reductase System Containing Cytochrome b(5) Type B (CYB5B) and MOSC2 Is of Importance for Lipid Synthesis in Adipocyte Mitochondria. J. Biol. Chem. 287 , 6307–6317 (2012).
Zheng, J., Cao, Y., Yang, J. & Jiang, H. UBXD8 mediates mitochondria-associated degradation to restrain apoptosis and mitophagy. EMBO Rep. 23 , e54859 (2022).
Ganji, R. et al. The p97-UBXD8 complex regulates ER-Mitochondria contact sites by altering membrane lipid saturation and composition. Nat. Commun. 14 , 638 (2023).
Baburina, Y. et al. Mitochondrial 2’, 3’-cyclic nucleotide 3’-phosphodiesterase (CNP) interacts with mPTP modulators and functional complexes (I-V) coupled with release of apoptotic factors. Neurochem. Int. 90 , 46–55 (2015).
Olga, K., Yulia, B. & Vassilios, P. The Functions of Mitochondrial 2’,3’-Cyclic Nucleotide-3’-Phosphodiesterase and Prospects for Its Future. Int J. Mol. Sci. 21 , 3217 (2020).
De, R. et al. Macrophage migration inhibitory factor regulates mitochondrial dynamics and cell growth of human cancer cell lines through CD74-NF-kappaB signaling. J. Biol. Chem. 293 , 19740–19760 (2018).
Yang, P. et al. Tip60- and sirtuin 2-regulated MARCKS acetylation and phosphorylation are required for diabetic embryopathy. Nat. Commun. 10 , 282 (2019).
Read, T. A. et al. The actin binding protein profilin 1 is critical for mitochondria function. bioRxiv , https://doi.org/10.1101/2023.1108.1107.552354 (2023).
Uezu, A. et al. Identification of an elaborate complex mediating postsynaptic inhibition. Science 353 , 1123–1129 (2016).
Dumrongprechachan, V. et al. Cell-type and subcellular compartment-specific APEX2 proximity labeling reveals activity-dependent nuclear proteome dynamics in the striatum. Nat. Commun. 12 , 4855 (2021).
Takano, T. et al. Chemico-genetic discovery of astrocytic control of inhibition in vivo. Nature 588 , 296–302 (2020).
Ke, M. et al. Spatiotemporal profiling of cytosolic signaling complexes in living cells by selective proximity proteomics. Nat. Commun. 12 , 71 (2021).
Wang, J. H. et al. Characterization of protein unfolding by fast cross-linking mass spectrometry using di-ortho-phthalaldehyde cross-linkers. Nat. Commun. 13 , 1468 (2022).
Zhai, Y. et al. Spatiotemporal-resolved protein networks profiling with photoactivation dependent proximity labeling. Nat. Commun. 13 , 4906 (2022).
Zheng, F., Yu, C., Zhou, X. & Zou, P. Genetically encoded photocatalytic protein labeling enables spatially-resolved profiling of intracellular proteome. Nat. Commun. 14 , 2978 (2023).
Bogdanow, B. et al. Spatially resolved protein map of intact human cytomegalovirus virions. Nat. Microbiol 8 , 1732–1747 (2023).
Wieckowski, M. R., Giorgi, C., Lebiedzinska, M., Duszynski, J. & Pinton, P. Isolation of mitochondria-associated membranes and mitochondria from animal tissues and cells. Nat. Protoc. 4 , 1582–1590 (2009).
Huttner, W. B., Schiebler, W., Greengard, P. & De Camilli, P. Synapsin I (protein I), a nerve terminal-specific phosphoprotein. III. Its association with synaptic vesicles studied in a highly purified synaptic vesicle preparation. J. Cell Biol. 96 , 1374–1388 (1983).
Takamori, S. et al. Molecular anatomy of a trafficking organelle. Cell 127 , 831–846 (2006).
Mick, D. U. et al. MITRAC links mitochondrial protein translocation to respiratory-chain assembly and translational regulation. Cell 151 , 1528–1541 (2012).
Liu, F., Lossl, P., Scheltema, R., Viner, R. & Heck, A. J. R. Optimized fragmentation schemes and data analysis strategies for proteome-wide cross-link identification. Nat. Commun. 8 , 15473 (2017).
Stephan, T., Roesch, A., Riedel, D. & Jakobs, S. Live-cell STED nanoscopy of mitochondrial cristae. Sci. Rep. 9 , 12419 (2019).
Krogh, A., Larsson, B., von Heijne, G. & Sonnhammer, E. L. Predicting transmembrane protein topology with a hidden Markov model: application to complete genomes. J. Mol. Biol. 305 , 567–580 (2001).
Download references
Acknowledgements
The work was funded by Deutsche Forschungsgemeinschaft Grant (DFG) SFB 958(Z03), DFG Project LI 3260/5-1, DFG Project LI 3260/6-1, the Leibniz-Wettbewerb (K284/2019 and P70/2018), and ERC-2020-StG (project number 949184) to F.L. as well as an FMP integrative project to M.L. and F.L.
Open Access funding enabled and organized by Projekt DEAL.
Author information
Authors and affiliations.
Department of Structural Biology, Leibniz-Forschungsinstitut für Molekulare Pharmakologie (FMP), Robert-Roessle-Str. 10 13125, Berlin, Germany
Ying Zhu, Kerem Can Akkaya, Julia Ruta, Nanako Yokoyama, Cong Wang, Max Ruwolt, Diogo Borges Lima & Fan Liu
Department of Molecular Physiology and Cell Biology, Leibniz-Forschungsinstitut für Molekulare Pharmakologie (FMP), Robert-Roessle-Str. 10 13125, Berlin, Germany
Kerem Can Akkaya & Martin Lehmann
Charité – Universitätsmedizin Berlin, Charitépl. 1, 10117, Berlin, Germany
You can also search for this author in PubMed Google Scholar
Contributions
Y.Z. performed most of the experiments and data analysis. K.A. performed the confocal and STED imaging experiments. C.W., K.A. and M.R. contributed to XL-MS data generation; J.R. developed the python script for CLASP automation. D.B.L., C.W., and N.Y. assisted the experiments and data analysis. M.L. supervised the imaging analysis. Y.Z. and F.L. wrote the manuscript. F.L. developed the concept and supervised the research. All authors reviewed and edited the manuscript.
Corresponding author
Correspondence to Fan Liu .
Ethics declarations
Competing interests.
F.L. is a shareholder and advisory board member of Absea Biotechnology Ltd. and VantAI. The remaining authors declare no competing interests.
Peer review
Peer review information.
Nature Communications thanks the anonymous reviewer(s) for their contribution to the peer review of this work. A peer review file is available.
Additional information
Publisher’s note Springer Nature remains neutral with regard to jurisdictional claims in published maps and institutional affiliations.
Supplementary information
Supplementary information, peer review file, description of additional supplementary files, supplementary data 1-11, reporting summary, source data, source data, rights and permissions.
Open Access This article is licensed under a Creative Commons Attribution 4.0 International License, which permits use, sharing, adaptation, distribution and reproduction in any medium or format, as long as you give appropriate credit to the original author(s) and the source, provide a link to the Creative Commons licence, and indicate if changes were made. The images or other third party material in this article are included in the article’s Creative Commons licence, unless indicated otherwise in a credit line to the material. If material is not included in the article’s Creative Commons licence and your intended use is not permitted by statutory regulation or exceeds the permitted use, you will need to obtain permission directly from the copyright holder. To view a copy of this licence, visit http://creativecommons.org/licenses/by/4.0/ .
Reprints and permissions
About this article
Cite this article.
Zhu, Y., Akkaya, K.C., Ruta, J. et al. Cross-link assisted spatial proteomics to map sub-organelle proteomes and membrane protein topologies. Nat Commun 15 , 3290 (2024). https://doi.org/10.1038/s41467-024-47569-x
Download citation
Received : 04 March 2023
Accepted : 05 April 2024
Published : 17 April 2024
DOI : https://doi.org/10.1038/s41467-024-47569-x
Share this article
Anyone you share the following link with will be able to read this content:
Sorry, a shareable link is not currently available for this article.
Provided by the Springer Nature SharedIt content-sharing initiative
By submitting a comment you agree to abide by our Terms and Community Guidelines . If you find something abusive or that does not comply with our terms or guidelines please flag it as inappropriate.
Quick links
- Explore articles by subject
- Guide to authors
- Editorial policies
Sign up for the Nature Briefing newsletter — what matters in science, free to your inbox daily.

Toward Pharmacy-Led Value Based Care Model for Buprenorphine Initiation and Management (PharmValue): Development of a Scalable Pharmacist-Led Collaborative Practice Agreement for Medication for Opioid Use Disorder
Pharmacist involvement in managing medications for opioid use disorder (MOUD), such as buprenorphine, has been previously shown feasible and has the potential for nationwide scalability. To maximize impact, such models must make use of scope of practice enhancing authorities, such as collaborative practice agreements. This project will: (1) engage community stakeholders to co-develop a model collaborative practice agreement for pharmacist-managed buprenorphine care and (2) identify existing legal authorities and advocacy opportunities to expand pharmacist-managed buprenorphine care in all 50 states. This will be accomplished using a community-engaged research approach as well as two 50-state reviews of state statutes, rules, and adopted policies or existing guidelines related to pharmacist-managed MOUD, particularly focused on pharmacist prescriptive authority and, separately, existing state-level legal barriers to MOUD care.
Principal Investigator(s)
Kenneth hohmeier, pharmd.
881 Madison Avenue Memphis , TN 38163 United States
Rachel Barenie, PharmD, J.D., M.P.H.
- Member Login
CompScope™ Benchmarks, 24th Edition
By rebecca (rui) yang, carol a. telles, evelina radeva, bogdan savych, karen rothkin, roman dolinschi, william monnin-browder.
April 23, 2024 Related Topics: CompScope™ Benchmarks
These comprehensive reference reports measure the performance of 17 state workers’ compensation systems, how they compare with each other, and how they have changed over time.
The reports are designed to help policymakers and others benchmark state system performance or a company’s workers’ compensation program. The benchmarks also provide an excellent baseline for tracking the effectiveness of policy changes and identifying important trends, including the impact of COVID-19.
The reports examine how income benefits, overall medical payments, costs, use of benefits, duration of temporary disability, litigiousness, benefit delivery expenses, timeliness of payments, and other metrics of system performance have changed from 2017 through 2022, with claims experience through 2023.
The 17 states in the study are Arkansas, California , Florida , Illinois , Indiana , Iowa, Louisiana , Massachusetts , Michigan , Minnesota , New Jersey , North Carolina , Pennsylvania , Tennessee, Texas , Virginia , and Wisconsin . There are individual reports for every state except Arkansas, Iowa, and Tennessee.
Note that the results we report reflect experience on claims through March 2023, including non-COVID-19 claims from the three years since the COVID-19 pandemic began. The study, therefore, provides a look at how the pandemic impacted non-COVID-19 workers’ compensation claims in the first three years of the pandemic.
CompScope™ Benchmarks, 24th Edition. Roman Dolinschi, William Monnin-Browder, Evelina Radeva, Karen Rothkin, Bogdan Savych, Carol A. Telles, and Rebecca Yang. April 2024. WC-24-01 to WC-24-14.
Copyright: WCRI
Share this report
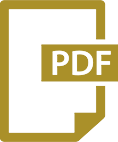
pdf, 399 KB
Reports are free for members.
If you are a member, please login here
*Temporary membership will allow you to download the study represented here
Research Questions:
- How does the performance of a state workers’ compensation system compare with that of other states?
- How has the performance of a state system changed over time?
- What has been the impact of the COVID-19 pandemic and changes in the economic environment on state workers' compensation systems?
Contact WCRI
To obtain your member login or to answer any questions or concern you may have, please contact us here.
- What is your question about? Select Membership Press Website Research Other
- Describe question
- Submit form Submit
FREE REPORT
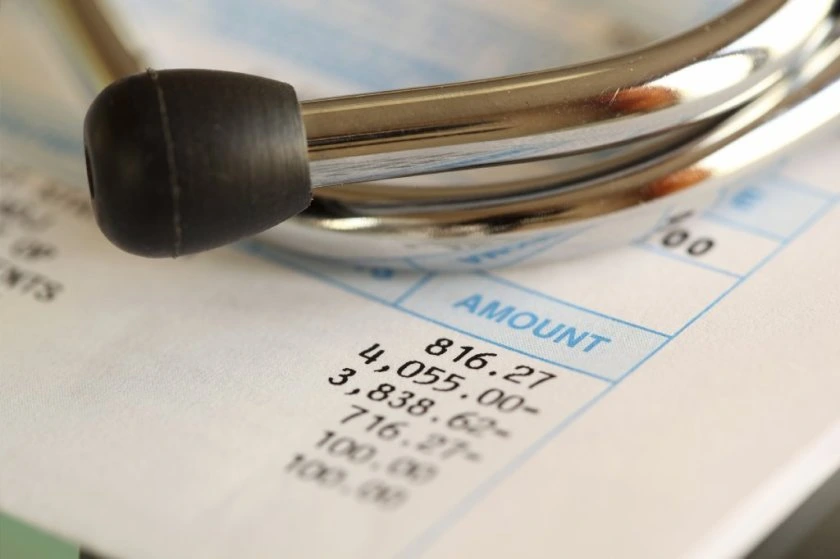
The increasing costs of medical care for treating injured workers have been a focus of public policymakers and system stakeholders in many states. To help them conduct meaningful comparisons of prices paid across states, and to monitor the price trends in relation to changes in fee schedules and network participation, this annual study creates an index for the actual prices paid for professional services across based on a marketbasket of commonly used services for treating injured workers.
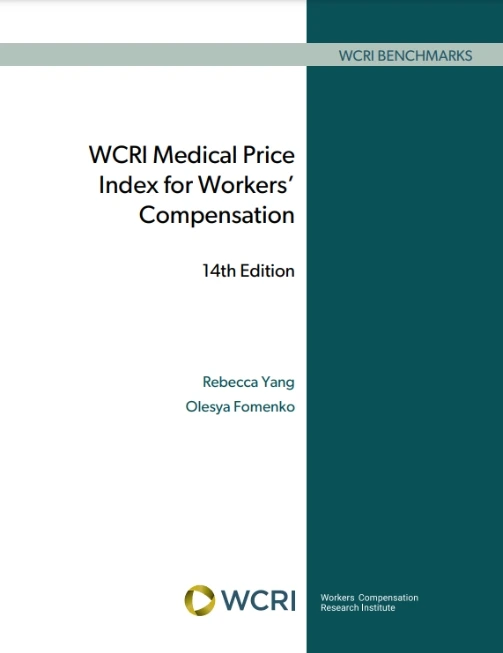
The increasing costs of medical care for treating injured workers have been a focus of public policymakers and system stakeholders in many states. Download our 31-state study to compare medical prices paid across states and to monitor trends in relation to policy choices and changes in fee schedules.
Research on Static Behavior of Stud Shear Connectors after Strong-corrosion Based on Push-out Test
- Structural Engineering
- Published: 17 June 2023
- Volume 27 , pages 3418–3429, ( 2023 )
Cite this article
- Wenjing Qiao ORCID: orcid.org/0000-0002-1832-1166 1 ,
- Qihan Hu ORCID: orcid.org/0000-0002-0245-7560 1 ,
- Fan Yang ORCID: orcid.org/0000-0001-7734-5224 1 &
- Haoyun Zhu 2
75 Accesses
Explore all metrics
To study the shear bearing performance and failure mechanism of stud shear connectors after strong-corrosion, six groups of stud shear connectors were designed and then welded to the specimen. They are corroded by hydrochloric acid time for 0 h, 2 h, 12 h, 24 h, 48 h, and 72 h. Herein, the loading process, load-slip curve, shear bearing capacity, and failure mode were obtained by the push-out test. The bearing capacity formula was also proposed by the stress mechanism of 84 finite element models. The results of the study on stud shear connectors show that the shear capacity, deformation capacity and slip decrease gradually with the extension of corrosion time. Among them, the enlargement in the diameter exerts considerable influence on their shear capacity. Notwithstanding, the shear bearing capacity slightly increases with stud shear connectors lengthened. The concrete strength grade can strengthen the shear capacity. Finally, the shear bearing capacity formula, taking the reliability coefficient into account, is proposed by the exit test data of 104 stud shear connectors.
This is a preview of subscription content, log in via an institution to check access.
Access this article
Price includes VAT (Russian Federation)
Instant access to the full article PDF.
Rent this article via DeepDyve
Institutional subscriptions
Similar content being viewed by others
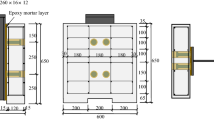
Experimental Study of the New Type Stud Shear Connector for Assembled Steel–Concrete Beams
Senqiang Lu, Wei Zhao & Zhenzhu Guo
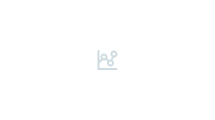
Comparison of Static and Fatigue Behaviors between Stud and Perfobond Shear Connectors
Bing Wang, Qiao Huang & Xiaoling Liu
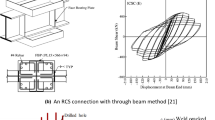
Push-Out Behavior of Encased Rigid Shear Connectors in Steel–Concrete Joints
Mohammad Mehdi Ahmadi, Seyed Rasoul Mirghaderi & Nasrin Bakhshayesh Eghbali
AISC (2016) 341-16, Seismic provisions for structural steel buildings Chicago (IL). American Institute for Steel Construction
Cao JH, Shao XD (2019) Finite element analysis of headed studs embedded in thin UHPC. Journal of Construction Steel Research 161:355–368, DOI: https://doi.org/10.1016/j.jcsr.2019.03.016
Article Google Scholar
Cao C, Zheng SS, Hu WB (2019) A survey on concrete structure properties under acid rain erosion. Material Reports 33(11):1869–1874, DOI: https://doi.org/10.11896/cldb.17110051
Google Scholar
Chen H, Guo ZX, Liu Y, Guo LT (2019) Study on the shear resisting mechanism and strength for an innovative composite shear connector. Engineering Mechanics 36(3):159–168, DOI: https://doi.org/10.6052/j.issn.1000-4750.2018.01.0058
Chen J, Zhao YX, Wu L, Jin WL (2016) Experimental investigation and design of corroded stud shear connectors. Advances in Structural Engineering 19(2):218–226, DOI: https://doi.org/10.1177/1369433215624327
Dao DK, Nguyen DK, Kim HS, Jeong YS, Kim IT (2018) A time-dependent corrosion characteristic of a steel member in contact with concrete. International Journal of Steel Structure 10(3):976–992, DOI: https://doi.org/10.1007/s13296-018-0037-7
Deng L, Ning SL, Wang W (2019) Calculation of the fatigue life of steel-concrete composite girder bridges under corrosive environment. Highway Engineering 44(2):97–102, DOI: https://doi.org/10.19782/j.cnki.1674-0610.2019.02.018
Ding FX, Ni M, Gong YZ, Yu ZW, Zhou Z, Zhou LC (2014) Experimental study on slip behavior and calculation of shear bearing capacity for shear stud shear connectors. Journal of Build Structure 35(9):98–106, DOI: https://doi.org/10.14006/j.jzjgxb.2014.09.013
Dong HY, Zhao YZ, Cao WL, Chen X, Zhang J (2021) Interfacial bond-slip behaviour between reinforced high-strength concrete and built-in steel plate with studs. Engineering Structures 226(3):11–17. DOI: https://doi.org/10.1016/j.engstruct.2020.111317
Fang H, Yang K, Du X, Li B, Zhang X, Tan P (2020) Experimental study on the mechanical properties of corroded concrete pipes subjected to diametral compression. Construction and Build Material 261:120576, DOI: https://doi.org/10.1016/j.conbuildmat.2020.120576
Křivý V Kubzová M, Konečnü P, Kreislová K (2019) Corrosion processes on weathering steel bridges influenced by deposition of deicing salts. Material 12(7):1120–1131, DOI: https://doi.org/10.3390/ma12071089
Lee PG, Shim CS, Chang SP (2005) Static and fatigue behavior of large stud shear connectors for steel-concrete composite bridges. Journal of Construction Steel Research 61(9):1270–1285, DOI: https://doi.org/10.1016/j.jcsr.2005.01.007
Ollgaard JG, Slutter RQ Fisher JW (1997) Shear strength of stud shear connectors in lightweight and normal-weight concrete. Engineer Journal of AISC 8(2):55–64
Qiao WJ, Yang F, Hu QH, Zhang H, Jiao XF (2023) Experiment on mechanical property degradation of Q345 steel after strong corrosion. Journal of Traffic and Transportation Engineering 1–14, DOI: https://doi.org/10.19818/j.cnki.1671-1637.2022.05.014
Qiao WJ, Zhang H, Zhang G, Yang F, Liu Z (2022) Tensile-mechanical degradation-properties and J-C constitutive model of studs after strong-acid corrosion. KSCE Journal of Civil Engineering 1–14, DOI: https://doi.org/10.1007/s12205-022-1438-x
Qiao WJ, Zhu HY, Zhang Q Yang F, Zhang H (2021) Mechanical properties and failure mechanism of steel plate composite beams under strong corrosion. Journal of Chang’an University (Natural Science Edition) 41(02):46–54, DOI: https://doi.org/10.19721/j.cnki.1671-8879.2021.02.005
Shi WH (2013) Mechanical behavior and reliability of steel-concrete composite beams considering durability. PhD Thesis, Central South University, Changsha, China (in Chinese)
Song C, Zhang G, Kodur V, Zhang Y, He S (2021) Fire response of horizontally curved continuous composite bridge girders. Journal of Constructional Steel Research 182:106671, DOI: https://doi.org/10.1016/j.jcsr.2021.106671
Song RN, Zhan YL, Zhao RD, Mou TM, Fan BK, Shao JH (2019) Push-out test and numerical analysis of embedded shear connectors with corrugated steel plates. China Journal of Highway and Transport 32(5): 88–99, DOI: https://doi.org/10.19721/j.cnki.1001-7372.2019.05.009
Tong LW, Chen LH, Wen M, Xu C (2020) Static behavior of stud shear connectors in high-strength-steel–UHPC composite beams. Engineering Structures 218:1–15, DOI: https://doi.org/10.1016/j.engstruct.2020.110827
Wang B (2013) Study on residual mechanical properties of steel-concrete composite beam bridges based on cumulative fatigue damnification. PhD Thesis, Southeast University, Nanjing, China (in Chinese)
Wang WH (2018) Experimental and analytical study on shear properties of headed stud connector. PhD Thesis, Zhejiang University, Zhejiang, China (in Chinese)
Wang JQ, Qi JN, Tong T, Xu Q, Xiu H (2018) Static behavior of large stud shear connectors in steel-UHPC composite structures. Engineering Structures 178(1):534–542, DOI: https://doi.org/10.1016/j.engstruct.2018.07.058
Wang CS, Wang YZ, Cui B, Qu TY, Sun YJ (2017) Experiment on effect of stress ratio on out-of-plane distortion induced fatigue performance of web gaps in steel bridges. China Journal of Highway and Transport 30(3):72–81, DOI: https://doi.org/10.19721/j.cnki.1001-7372.2017.03.008
Xue W, Chen J, Zhu JH (2017) Behaviour of corroded single stud shear connectors. Material 10(3):276, DOI: https://doi.org/10.3390/ma10030276
Xue WC, Ding M, Wang H, Luo Z (2008) Static behavior and theoretical model of stud shear connectors. Journal of Bridge Engineering 13(6):623–634, DOI: https://doi.org/10.1061/(ASCE)1084-0702(2008)13:6(623)
Yang F, Zhu HY, Qiao WJ, Du B (2022) The load-slip characteristics of stud shear connector after hydrochloric acid corrosion. Structures 41:463–474, DOI: https://doi.org/10.1016/j.istruc.2022.05.039
Zhang H, Qiao WJ, Yang F, Zhu HY (2021) Tensile finite element simulation of Q345 bridge steel with strong corrosion based on J-C model. Journal of Zhengzhou University (Engineering Science) 42(6):99–104, DOI: https://doi.org/10.13705/j.issn.1671-6833.2021.04.021
Zheng SJ, Liu Y, Yoda T, Lin W (2016) Parametric study on shear capacity of circular-hole and long-hole perfobond shear connector. Journal of Construction Steel Research 117:64–80, DOI: https://doi.org/10.1016/j.jcsr.2015.09.012
Zheng KF, Zheng Y, Heng JL, Wang YW (2020) High strength weathering steel and its application and prospect in bridge engineering. Journal of Harbin Institute of Technology 52(3):1–10, DOI: https://doi.org/10.11918/201907021
Zhu JS, Guo XY, Kang JF, Huo HX (2019) Research on corrosion behavior, mechanical property and application of weathering steel in bridges. China Journal of Highway and Transport 32(5):1–16, DOI: https://doi.org/10.19721/j.cnki.1001-7372.2019.05.001
Download references
Acknowledgments
This work was supported by Education Department Project of Shaanxi Province (Grant No. 20JK0678, 21JK0828), Science and technology innovation Team of Shaanxi Province (Grant No. 2023-CX-TD-38), Science Foundation for Distinguished Young Scholars of Shaanxi Province (Grant No. 2022JC-23).
Author information
Authors and affiliations.
Civil & Architecture Engineering, Xi’an Technological University, Xi’an, 710021, China
Wenjing Qiao, Qihan Hu & Fan Yang
Shaanxi Province Traffic Planning Design Institute, Xi’an, 710000, China
You can also search for this author in PubMed Google Scholar
Corresponding author
Correspondence to Wenjing Qiao .
Rights and permissions
Reprints and permissions
About this article
Qiao, W., Hu, Q., Yang, F. et al. Research on Static Behavior of Stud Shear Connectors after Strong-corrosion Based on Push-out Test. KSCE J Civ Eng 27 , 3418–3429 (2023). https://doi.org/10.1007/s12205-023-1359-3
Download citation
Received : 29 July 2022
Accepted : 13 April 2023
Published : 17 June 2023
Issue Date : August 2023
DOI : https://doi.org/10.1007/s12205-023-1359-3
Share this article
Anyone you share the following link with will be able to read this content:
Sorry, a shareable link is not currently available for this article.
Provided by the Springer Nature SharedIt content-sharing initiative
- Shear bearing performance
- Failure mechanism
- Push-out test
- Reliability analysis
- Strong-corrosion
- Find a journal
- Publish with us
- Track your research
- Support Dal
- Current Students
- Faculty & Staff
- Family & Friends
- Agricultural Campus (Truro)
- Halifax Campuses
- Campus Maps
- Brightspace
Dalhousie University
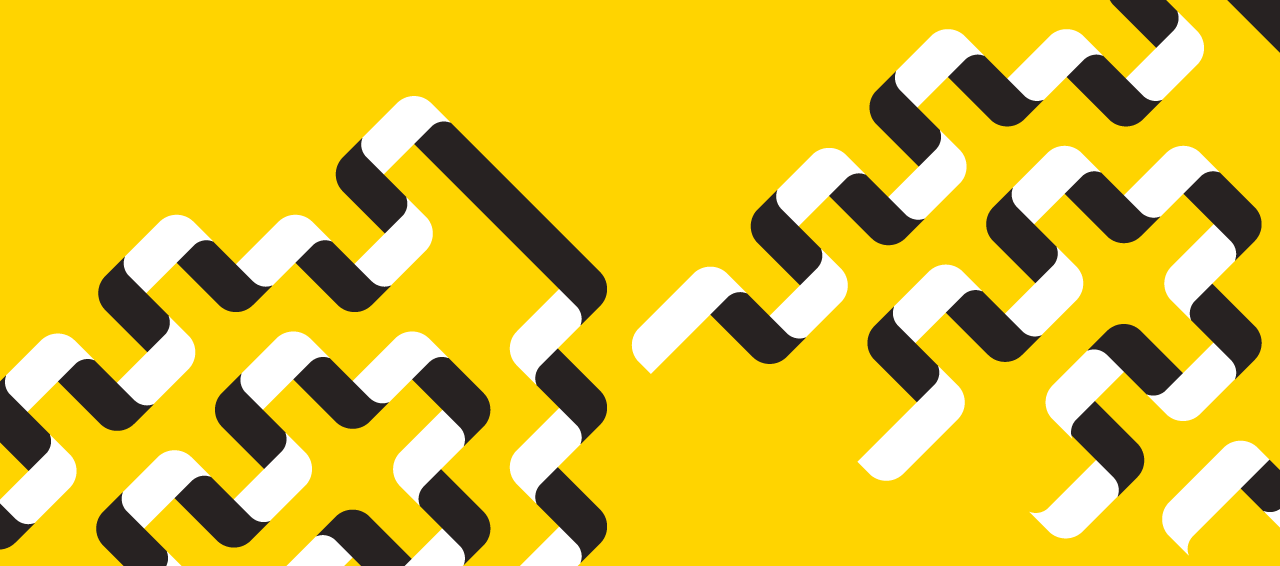
- Student Life
- Media Centre
- DAL Magazine
Most Commented
News archive.
- February 2024
- January 2024
- December 2023
- November 2023
- October 2023
Taking research to new places: Killam scholar trades Atlantic coast for expansive mountain ranges
Mia Samardzic - April 22, 2024
This article is part of a series profiling the inaugural Killam International Research Award recipients who traveled abroad in 2023.
This time last year, biology PhD student Sam Walmsley was carrying out his research on an endangered population of northern bottlenose whales found in waters close to Nova Scotia — but he was far from the Atlantic coast himself.
Walmsley was one of three recipients selected for the inaugural Killam International Research Award launched in the fall of 2022, which provides rich global experiences for exceptional Killam scholars by offsetting the costs of undertaking research outside of Canada for up to three months.
The award gave Walmsley the opportunity to travel to Zurich, Switzerland from March to May of last year, where he worked with professor Dr. Adrian Jaeggi, who leads a lab investigating the evolution and ecology of human behaviour.
Understanding whale societies
Walmsley’s research aims to uncover the evolutionary origins and functions of whale societies, which share some similarities with human societies that aren’t found in any other animal groups.
“Understanding why these similarities arose despite such different physical environments will allow us to explain the evolution of these traits across species,” he says.
“Studying whales can offer a unique window into the origins of social living, meaning that we can ask questions like 'Why do some species form friendships and not others?' or 'What prompts individuals to cooperate with non-relatives?' For whales specifically, understanding how individuals interact with one another and share knowledge can become important for conservation. For example, social patterns may affect a population’s susceptibility for disease, or influence risky group movements as seen in mass stranding events.”
The Killam International Research Award exposed Walmsley to cutting-edge quantitative tools developed by researchers at the University of Zurich, serving as a lens into evolutionary history and helping to explain the origins of social complexity.
“Though originally applied to human evolution, applying this method to whales allows us to ask new questions like 'Were ancient whale species solitary, or did they rely on others?' This allowed me to expand my research beyond Nova Scotian waters to compare and model societies from whale and dolphin species all around the world.”
International immersion
The opportunity to expand his research in a major way wasn’t the only highlight of Walmsley’s trip.
“It was thrilling to immerse myself in the academic environment at the University of Zurich and the cultural environment of Zurich itself. Outside of my studies, I was able to explore the local mountains, consume many delicious cheeses, and even float down the Limmat River in an inflatable boat with my lab mates one Sunday afternoon. I am enormously grateful to professor Jaeggi and the members of his lab who made it such an interesting and enjoyable experience,” he says.
Walmsley describes being a Killam Scholar as an incredible privilege, granting him the opportunity to explore ideas with global experts in his field.
“Applying the new methods I was exposed to in Zurich to whale populations allows me to expand the scope of my thesis and situate my study of northern bottlenose whales in a wider evolutionary context,” he says. “There is a growing recognition that human impacts can disrupt the social structure of wild animals, which in turn can have consequences for the survival of the population. By monitoring and learning from these whales, I hope that we can continue to ensure their protection for years to come.”
Learn more about the Killam advantage and opportunities at Dalhousie University.
Recommended reading: New Dal‑based Killam award opens (lab) door for tumour researcher (from the same series)
Dal News welcomes discussion from members of the Dalhousie community and beyond, but urge comment writers to be respectful and refrain from personal attacks. False or unsubstantiated allegations, libellous statements and offensive language are not allowed. External links must be appropriate and relevant to the subject being discussed.
We encourage commenters to use their real first and last names.
Please note that comments that appear on the site are not the opinion of Dal News or Dalhousie University but only of the comment writer. The editors reserve the right to post, or not to post comments, edit or not edit, at their discretion.
Halifax, Nova Scotia, Canada B3H 4R2 1-902-494-2211
Agricultural Campus Truro, Nova Scotia, Canada B2N 5E3 1-902-893-6600
- Campus Directory
- Student Career Services
- Employment with Dalhousie
- For Parents
- For Employers
- Privacy Statement
- Terms of Use
Dalhousie University Halifax, Nova Scotia, Canada B3H 4R2 1.902.494.2211
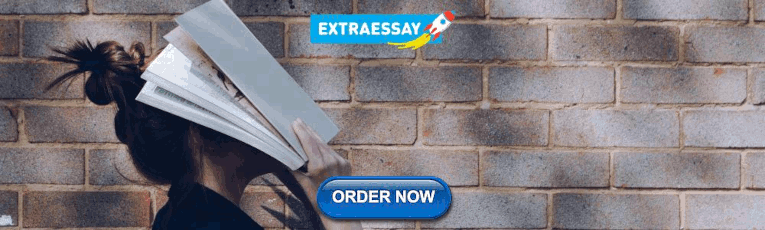
IMAGES
VIDEO
COMMENTS
The scope of the study is the boundaries within which your research project will be performed. It defines the aspects that will be considered and those that will not be considered in your research. Learn why it is important, how to write it and see an example of a scope of a study for a research question on COVID-19 mental health.
Scope of research refers to the range of topics, areas, and subjects that a research project intends to cover. It is the extent and limitations of the study, defining what is included and excluded in the research. The scope of a research project depends on various factors, such as the research questions, objectives, methodology, and available ...
Your study's scope and delimitations are the sections where you define the broader parameters and boundaries of your research. The scope details what your study will explore, such as the target population, extent, or study duration. Delimitations are factors and variables not included in the study. Scope and delimitations are not methodological ...
Scope of research is the set of variables, criteria, or conditions that you choose to include or exclude in your project. Learn how to define your scope of research for different types of research, such as quantitative, qualitative, and mixed methods, and why it is important for your goals and outcomes.
Learn how to write a scope and delimitations statement for your research paper or thesis. Find out the difference between scope and limitations, and how to formulate them based on your research question, aims, objectives, variables, population, timeframe and methods. See examples of scope and delimitations in research.
Answer: The scope of a study explains the extent to which the research area will be explored in your work, and it specifies the parameters within which the study will be operating. In other words, you will have to define what the study will cover and what it focuses on. Similarly, you also have to explain what the study will not cover.
The scope of your project sets clear parameters for your research.. A scope statement will give basic information about the depth and breadth of the project. It tells your reader exactly what you want to find out, how you will conduct your study, the reports and deliverables that will be part of the outcome of the study, and the responsibilities of the researchers involved in the study.
Example 1. Research question: What are the effects of social media on mental health? Scope: The scope of the study will focus on the impact of social media on the mental health of young adults aged 18-24 in the United States. Delimitation: The study will specifically examine the following aspects of social media: frequency of use, types of social media platforms used, and the impact of social ...
Scope of research is determined at the beginning of your research process, prior to the data collection stage. Sometimes called "scope of study," your scope delineates what will and will not be covered in your project. It helps you focus your work and your time, ensuring that you'll be able to achieve your goals and outcomes. ...
In academic research, defining the scope and delimitations is a pivotal step in designing a robust and effective study. The scope outlines the breadth and depth of the investigation, offering a clear direction for the research. Meanwhile, delimitations set the boundaries of the study, ensuring that the research remains focused and manageable.
Answer: Scope and delimitations are two elements of a research paper or thesis. The scope of a study explains the extent to which the research area will be explored in the work and specifies the parameters within which the study will be operating. For example, let's say a researcher wants to study the impact of mobile phones on behavior ...
A research design is a strategy for answering your research question using empirical data. Creating a research design means making decisions about: Your overall research objectives and approach. Whether you'll rely on primary research or secondary research. Your sampling methods or criteria for selecting subjects. Your data collection methods.
The scope of a study explains the extent to which the research area will be explored in the work and specifies the parameters within the study will be operating. Learn how to write an engaging and clear scope for your research paper with this Q&A from Editage Insights, a platform for researchers and authors.
Any study that describes or analyzes methods (design, conduct, analysis or reporting) in published (or unpublished) literature is a methodological study. Consequently, the scope of methodological studies is quite extensive and includes, but is not limited to, topics as diverse as: research question formulation ; adherence to reporting ...
The scope of a research paper explains the context and framework for the study, outlines the extent, variables, or dimensions that will be investigated, and provides details of the parameters within which the study is conducted. Delimitations in research, on the other hand, refer to the limitations imposed on the study.
To write your scope of the study, you need to restate the research problem and objectives of your study. You should state the period in which your study focuses on. The research methods utilized in your study should also be stated. This incorporates data such as sample size, geographical location, variables, and the method of analysis.
The scope of research delineates its extent or range of inquiry, setting clear parameters for what the study will cover. It's a foundational aspect that guides every step of the research process, from the formulation of research questions to the interpretation of results. Defining the scope helps in focusing the research efforts, ensuring ...
Delimitations refer to the specific boundaries or limitations that are set in a research study in order to narrow its scope and focus. Delimitations may be related to a variety of factors, including the population being studied, the geographical location, the time period, the research design, and the methods or tools being used to collect data.
1.5.3 The Research Purpose Statement. The purpose of the research is expressed as an objective of the study and usually identifies important elements, for example, variables, the site of the study, and the study population. In the paper by Seeram et al. ( 2016 ), the purpose statement is expressed as follows:
By Priya Chetty on January 23, 2020. The scope of the study refers to the elements that will be covered in a research project. It defines the boundaries of the research. The scope is always decided in the preliminary stages of a study. Deciding it in the later stages creates a lot of ambiguity regarding the research goals.
The scope of the study explains the extent to which your research area will be explored, and the parameters the study will operate. It gives the reader and the writer an insight into what the study is aimed at and what should be anticipated. This implies that the scope of the study should define the purpose of your study, the sample size and ...
This study extends the scope of cross-linking mass spectrometry beyond protein structure and interaction analysis towards spatial proteomics, and establishes a method for concomitant profiling of ...
To maximize impact, such models must make use of scope of practice enhancing authorities, such as collaborative practice agreements. This project will: (1) engage community stakeholders to co-develop a model collaborative practice agreement for pharmacist-managed buprenorphine care and (2) identify existing legal authorities and advocacy ...
The study, therefore, provides a look at how the pandemic impacted non-COVID-19 workers' compensation claims in the first three years of the pandemic. CompScope™ Benchmarks, 24th Edition. Roman Dolinschi, William Monnin-Browder, Evelina Radeva, Karen Rothkin, Bogdan Savych, Carol A. Telles, and Rebecca Yang. April 2024. WC-24-01 to WC-24-14.
To study the shear bearing performance and failure mechanism of stud shear connectors after strong-corrosion, six groups of stud shear connectors were designed and then welded to the specimen. They are corroded by hydrochloric acid time for 0 h, 2 h, 12 h, 24 h, 48 h, and 72 h. Herein, the loading process, load-slip curve, shear bearing capacity, and failure mode were obtained by the push-out ...
1 Answer to this question. Answer: The scope of a study explains the extent to which the research area will be explored in the study and specifies the parameters within which the study will be operating. Thus, the scope of a study will define the purpose of the study, the population size and characteristics, geographical location, the time ...
To have a robust clinical antifungal pipeline it's essential to invest and monitor its upstream development. In November 2022 WHO released the WHO fungal priority pathogens list (FPPL), a catalogue of the 19 fungi that represent the greatest threat to public health. The list is the first global effort to systematically prioritize fungal pathogens, considering the unmet research and development ...
The scope of a study establishes the extent to which you will study the topic in question. It's done to keep the study practical and relevant. The web page provides some examples of the scope of a study from different fields and topics, as well as tips on how to write it.
Biology PhD student Sam Walmsley travelled to Zurich, Switzerland last year after receiving the inaugural Killam International Research Award, an opportunity that enabled him to apply new methods to expand the scope of his study of northern bottlenose whales in a wider evolutionary context.