Systems, Synthetic, and Quantitative Biology
Share this page.
Harvard was one of the first institutions to offer a program to explore this exciting new field. The program’s core curriculum includes courses on the methods and logic that shape research, how to conceptualize and present research, and an introduction to the faculty’s research.
The program has 48 faculty located in the Faculty of Arts and Sciences, Harvard Medical School, and Harvard-affiliated teaching hospitals including Dana-Farber Cancer Institute, Mass General, and Boston Children’s Hospital. SSQB is one of 14 PhD programs in the Harvard Integrated Life Sciences program that collectively gives you access to over 900 faculty research groups situated in the heart of Boston’s biotech hub. Our students are working on projects that range from fundamental problems in biology to translational research, whose goal is to directly affect medicine and global sustainability.
Graduates of the program have gone on to faculty positions at prestigious institutions such as MIT and Princeton University, while others are now industry leaders as startup founders or as decision-makers at companies including Boston Consulting Group, Yumanity Therapeutics, McKinsey & Company, and Regeneron.
Additional information on the graduate program is available from the Systems, Synthetic, and Quantitative Biology PhD Program , and requirements for the degree are detailed in Policies .
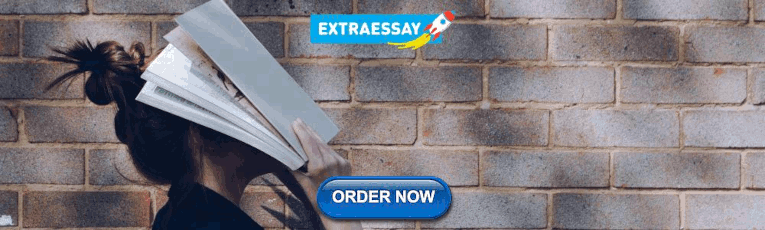
Admissions Requirements
Please review admissions requirements and other information before applying. You can find degree program-specific admissions requirements below and access additional guidance on applying from the Systems, Synthetic, and Quantitative Biology PhD Program .
Academic Background
Applicants typically have a background in biology, physics, chemistry, computer science, engineering, or mathematics and work to forge a new approach to biology that combines theoretical and experimental approaches. The typical student has a strong background in one of the disciplines relevant to systems biology and an interest in interdisciplinary research.
Standardized Tests
GRE General: Optional
Contacting Faculty
Applicants should indicate their faculty of interest in the application. You are not required to contact any faculty in advance but are welcome to.
Applications are reviewed by the admissions committee during December and early January. Selected applicants are notified if they have been chosen for an on-campus interview. These visits provide students with the opportunity to meet with faculty and current students and to get a better feel for our community and the types of research conducted here. Applicants invited for an interview who reside overseas and cannot visit the Harvard campus may interview remotely.
Theses & Dissertations
Theses & Dissertations for Systems, Synthetic, and Quantitative Biology
See list of Systems, Synthetic, and Quantitative Biology faculty
APPLICATION DEADLINE
Questions about the program.
St anford | Synthetic Biology
Enabling flourishing futures in partnership with life.
What is synthetic biology?
Synthetic Biology (SB) explores new forms of engagement with life and living systems. From molecular to ecological, cultural to political, SB is about understanding life's fundamental mysteries and translating knowledge to imagine a biotic civilization that flourishes in partnership with Earth.
The Stanford Synthetic Biology community enables interdisciplinary activities, supporting an ecosystem of research and learning. Our holistic approach encompasses diverse areas of work, each exploring fundamental questions and possibilities with the ultimate desire of addressing societal needs.
All together now!
Stewart Brand, Stanford Class of 19 60 (Biology), wrote,
"I propose six significant levels of pace and size in the working structure of a robust and adaptable civilization. [...] In a healthy society each level is allowed to operate at its own pace, safely sustained by the slower levels below and kept invigorated by the livelier levels above." ( Long Now )
With pace-layer thinking in mind we are exploring and advancing synthetic biology (SB) among and across all aspects of civilization, inclusive of but not limited to biotechnology and bioeconomy. We also note that intrinsic to pace layers thinking is a bias towards the human, a concept we suspect may evolve along with SB. Thus, we have adopted a coupled-rings visual metaphor, in place of layers, for now.
SB@ Stanford News
The Wu Tsai Neurosciences Institute , Sarafan Chem-H , and Bio-X seek proposals for the inaugural Synthetic Neuroscience Grant Program to spark new collaborations between neuroscience and synthetic biology researchers across Stanford University.
February 2024
Learn more and apply
Stanford Emerging Technology Review launch at the Hoover office in Washington, DC.
January 202 4
Watch the event recording
The second Synthetic Neuroscience Forum was held with talks from Michael Lin and Xiaojing Gao. Hosted by Sarafan ChEM-H, Bio-X, Wu Tsai Neurosciences Institute.
January 2024
Event Information
Building Biology student symposium brought in guest speakers: Patrick Hsu (UCB), Katie Galloway (MIT), Magdalena Zernika-Goetz (Caltech), Neil Shubin (UChicago)
Hosted by Stanford Genetics and Developmental Biology Training Program
January 2024
The Hoover Institution and Stanford School of Engineering have launched the inaugural edition of the Stanford Emerging Technology Review, which aims to help policymakers understand a range of fast-developing new technologies, from AI and cryptography to robotics and synthetic biology.
December 2023
Read the Report
The first Synthetic Neuroscience Forum was held with Drew Endy and Sergiu Pasca, leading a discussion on the opportunities of synthetic biology in neuroscience. Hosted by Sarafan ChEM-H, Bio-X, Wu Tsai Neurosciences Institute.
December 2023
Stanford Bioengineering Department launches "Engineering Life for Planet Health" f aculty search and seeking candidates who align with the department's vision of rapidly advancing large-scale solutions to address critical planetary challenges.
November 2023
Qi Lab develops an ultrasound method for precise control in gene regulation and base editing - both in cells and living animals.
October 2023
Read in Nature
Congratulations to Xiaojing Gao, Steven Banik, and Stanley Qi for being awarded NIH High Risk, High Reward program grants for their work in synthetic biology.
Read in Stanford Report
Mike Jewett kicks off the new school year by facilitating a community discussion at SB.Talk on synthetic biology for sustainability.
September 2023
Upcoming Events
Stanford 2023 iGEM team designed and developed Phil's Laberia, an educational video game where students can learn bioengineering wet lab skills.
August 2023
Read the article
Play the Game
Fischbach Lab provides new insights into how T cells respond to commensal microbes.
Qi Lab develops a CRISPR/Cas9 approach to edit dendritic cells for wound healing
Read in Nature Comms
Bintu Lab works on high-throughput functional characterization of combinations of transcriptional activators and repressors
Read in Cell Systems
Endy Lab publishes a paper on engineering tRNA abundances for synthetic cellular systems
Learn from Jenn Brophy and Drew Endy 's EdEquityLab x Stanford 's Intro to Bioengineering course. Now available online!
Watch the lectures
SB.Talk sparks discussion around synthetic biology at Stanford. Join the conversation in the Shriram Tea Room every other Tuesday 12pm.
Jewett Lab developed a cell-free method to rapidly discover and characterize functional antibodies in under 24 hours.
Qi Lab reports on 'CLIP' (CRISPR for long-fragment integration via pseudovirus) method for stable expression of large transgenes via the knock-in of an integrate-deficient lentivirus.
Prof. Alice Ting has been elected to the National Academy of Sciences. Ting is a Professor of Genetics, of Biology and, by courtesy, of Chemistry.
Read in NAS News
The inaugural Synthetic Biology for Sustainability Symposium had over 200 attendees representing across schools of Medicine, Engineering, Sustainability, and H&S.
Event Website
Read highlights in Stanford News
Synthetic Biology for Sustainability Symposium will take place on May 1.
Hosted by the Deans of the School of Medicine, the School of Engineering, and the Stanford Doerr School of Sustainability, this symposium will focus on how we might tackle some of the biggest challenges in sustainability using some of the newest innovations in synthetic biology.
Agenda and Speaker Information
Fischbach Lab engineered a common skin bacterium, S. epidermidis to produce a tumor antigen . When applied to mice, it resulted in a potent immune response against a distant tumor.
Read the Science article
Read in Stanford News
Bintu Lab leads efforts towards large-scale mapping and mutagenesis of human transcriptional effector domains
Read the Nature article
Stanford Synthetic Biology meets for the first community event of 2023
View our community portrait
Sattely Lab discovered 22 enzymes for biosynthesis of limonoids in Citrus and Melia.
January 2023
Prof. Mike Jewett joins Stanford Bioengineering as their newest faculty member.
Read the Stanford Article
Join the Jewett lab!
Gao Lab develops new tool -- programmable RNA sensing using ADAR editing in living cells.
October 2022
Read in Nature Biotechnology
Read the story!
Biden Administration issued an Executive Order on Advancing Biotechnology and Biomanufacturing Innovation for a Sustainable, Safe, and Secure American Bioeconomy.
September 2022
Learn more from Endy and Palmer
White House Executive Order
Does synthetic biology offer anything new? Watch the non-technical conversation with Drew Endy about advancements in synthetic biology and related economic and governance opportunities with t he Hoover Institution.
August 2022
Watch the video!
Sergiu Pasca explains how to reverse engineer the human brain by growing organoids.
August 2022
Learn from the TED talk!
Dr. Alice Cheng of Fischbach Lab & colleagues make human microbiome from scatch.
Read NY Times article
Study the science!
The Bintu lab takes a synthetic approach to understand and program chromatin-mediated gene silencing & activation.
Brophy et al. pioneer synthetic genetic circuits in plant roots.
Read the news!
Townshend, Kaplan, & Smolke report over 200 new RNA biosensors to potential drug molecules.
Read the preprint!
Endy & McKelvey reflect on synthetic biology's potential impacts for democracy and national security, hosted by Los Alamos National Lab. and moderated in part by Stanford's Prof. Hank Greely.
Synthetic Biology for Democracy
National Security Implications
Preparing for and preventing future pandemics. Two new grants provide key resources
Seed grants available Fall '22
Antiviral drug discovery funded!
"What is synthetic biology and what is its potential? These stories explain." Megan Palmer's WEF Council partners with Faber Futures to explore and elaborate on inclusive futures.
Download the stories!
"Building a Bottom-Up Bioeconomy," Stanford's Dr. Megan Palmer and colleagues make the case by reimagining industrialization .
Read the article!
Making With Mushrooms! 17 years later the second-ever issue of Adventures in Synthetic Biology begins to emerge.
Mycological mysteries await!
"Mother Nature, Bioweapons, & Lab Accidents: Guarding Against the Next Global Biological Catastrophe," Stanford's Freeman Spogli's CISAC welcomes Dr. Jaime Yassif of NTI. March 2022
View the presentation!
Prof. Pasca explores how to understand the mysteries of the human mind by growing neural circuits from scratch .
Train your neurons!
Prof. Skylar-Scott & team explain what must be made real to ultimately print working hearts from scratch.
"Who wouldn't want to ride on a Wooly Mammoth?," Stanford undergraduates explore dystopian and utopian futures that might arise via resurrecting extinct species. March 2022
Extinction Reversal Dystopia!
De-Extinction Utopia!
Prof. Ting's team pioneers LuCID, a genetically-encoded tool for realtime measurement of calcium dynamics in live cells, including neurons and immune cells.
Read the paper!
Prof. Steinmetz and colleagues pioneer synthetic genomics for understanding the fundamental rules of genome architecture.
Study the Science paper!
Read the news story!
The New Yorker explores if what biology needs right now is more synthesis. Prof. Zia chimes in.
February 2022
Prof. Brophy & Dinneny pioneer living Boolean logic in plants for climate resilience.
Study the preprint
Gao lab pioneers the engineering of protein circuits that let cells talk to each other.
Novozymes recognizes Adjunct Prof. Smolke & team with 100,000 DKK prize for sustainable medicines.
See the announcement!
How can American strengthen its bioeconomy? Adjunct Prof. Palmer and colleagues chart the path.
Read the plan!
Can we engineer crops to withstand climate change? Prof. Brophy makes the case.
January 2022
See the news!
NY Times explores what's happening and might be possible via synthetic biology. E.g., it's personal!
November 2021
Megan Palmer, co-chair of World Economic Forum Council on Synthetic Biology, and team release report on synthetic biology.
Read the report!
Danielle Mai's group discusses the role of synthetic biology in a class of protein-based materials.
September 202 1
Stanley Qi's team develops CRISPR-Cas13 resource for targeting RNA viruse s.
Prashanth in the Smolke lab pioneers brewing of tropane alkaloids from scratch.
September 2020
Read the coverage!
General Inquiries
Call today to schedule an appointment or fill out an online request form. If requested before 2 p.m. you will receive a response today.
713-798-1000
Monday - Friday 8 a.m. - 5 p.m.
Request Now
Request non-urgent appointments
Find a Physician
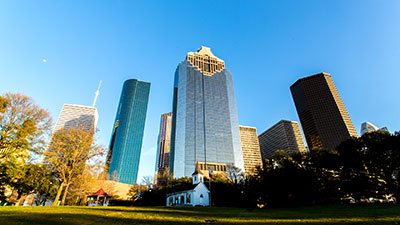
America's fourth largest city is a great place to live, work and play. Find out why. Get to Know Houston
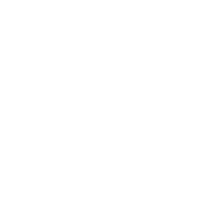
Give Careers Intranet
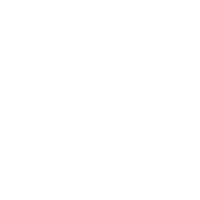
- Graduate School of Biomedical Sciences
- Degree Programs and Certificates
- Current Students
- Postdoctoral Affairs
- Faculty Mentoring Resources
- Connect with GSBS
- Graduate School Contacts
- Career Paths
- Student Life
- Training Grants
- Faculty & Staff
- Baylor College of Medicine
- Chemical, Physical & Structural Biology
Synthetic Biology
Synthetic biology is a multidisciplinary field that integrates molecular biology, genetics, systems biology, biotechnology, and biophysical approaches among many others. With the recent advances in bottom-up DNA assembly methods to stitch together and characterize adaptable functioning synthetic biological circuits, this field is becoming integral across biomedical research. Graduate students within the CPSB program are exposed to a variety of aspects of synthetic biology that aid them in designing and engineering biological systems useful in research areas as versatile as signal transduction, virology, metabolism, medicinal chemistry, structural biology, human disease modeling, and many others.
Faculty Profiles: Synthetic Biology

Graduate School Admissions
Find all the information you need to apply to the Baylor College of Medicine Graduate School of Biomedical Sciences.
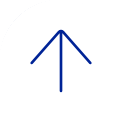
MIT Department of Biological Engineering
Search form.
- DEI Collaborative
- DEI Current Efforts
- DEI Newsletter
- Learning Resources
- Faculty Directory
- Staff Directory
- Open Faculty Positions
- Prospective Undergraduate
- Major Degree Requirements
- Minor Programs
- Undergraduate Thesis
- Research Prize
- BE Student Life
- Career Resources
- Master's Degree
- Graduate PhD Application
- Application Assistance Program
- Graduate FAQ
- Graduate Life
- Meet The Graduate Students
- PhD Course Requirements
- Advisor Selection
- PhD Written Exam
- Thesis Committee
- PhD Oral Exam
- PhD Dissertation Requirements
- BE Graduate Student Board
- Teaching Assistant Award Winners
- BE Communication Lab
- Research Areas
- Wishnok Prize
- BATS Resources
- BATS Archive
- For Undergraduate Students
- Professional Development
- For Post Docs
- Covid-19 Resources
- Laboratory Safety
- Faculty & Instructors
Ron Weiss, PhD
Lab website: , email: , office: , phone: , administrative assistant: , courses: , research-at-a-glance: , biography: .
Prof. Weiss is one of the pioneers of synthetic biology. He has been engaged in synthetic biology research since 1996 when he was a graduate student at MIT and where he helped set up a wet-lab in the Electrical Engineering and Computer Science Department. After completion of his PhD, Weiss joined the faculty at Princeton University, and recently returned to MIT to take on a tenured faculty position in the Department of Biological Engineering and the Department of Electrical Engineering and Computer Science.
Research:
Cells sense their environment, process information, and continuously react to both internal and external stimuli. The construction of synthetic gene networks can help improve our understanding of such naturally existing regulatory functions within cells. Synthetic gene networks will also enable a wide range of new programmed cells applications. The Weiss lab uses computer engineering principles of abstraction, composition, and interface specifications to program cells with sensors and actuators precisely controlled by analog and digital logic circuitry. Here, recombinant DNA-binding proteins represent signals, and recombinant genes perform the computation by regulating protein expression. The group constructed synthetic gene networks that implement biochemical logic circuits in Escherichia coli fabricated using the AND, NOT, and IMPLIES logic gates. The lab has built a variety of circuits, including a transcriptional cascade whose digital behavior improves significantly with the addition of genetic components. The group has also built analog circuits that perform signal processing to detect specific chemical gradients and generate pulses in response to cell-cell communication. The integration of digital and analog circuitry is useful for controlling the behavior of individual cells and the lab has also combined these circuits with engineered cell-cell communication to coordinate the behavior of cell aggregates.
Research Areas:
- Computational Modeling
- Microbial Systems
- Synthetic Biology
- Systems Biology
- Tissue Engineering

Synthetic Biology and Biological Design

Cover Image Credit: Keating Lab
Image Credit: Lu Lab
Research in Synthetic Biology and Biological Design emphasizes elucidating engineering principles behind biological systems for creating novel therapeutics and biomaterials.
Scientists track evolution of microbes on the skin’s surface
A new analysis reveals how Staphylococcus aureus gains mutations that allow it to colonize eczema patches .

Brandon (Brady ) Weissbourd
Katie galloway, lindsay case, hacking life inside and outside the laboratory.

Managing her own synthetic biology project helped graduate student Jesse Tordoff overcome imposter syndrome and hit her stride.
Anders Sejr Hansen

- Skip to primary navigation
- Skip to main content
- Skip to primary sidebar
- Skip to footer
UC Berkeley Department of Bioengineering
The future of biology. The future of engineering.
research_head

Systems & Synthetic Biology
Systems biology approaches living systems as interactive, multifaceted networks rather than as a collection of individual units. Synthetic biology seeks to build parts, devices, and systems from biological components. The goals of these efforts can include using microorganisms to synthesize materials of medical or industrial value, and even to repurpose bacteria to fight disease.
Efforts within systems biology focus on collection, curation, and analysis of large data sets, including genomic and proteomic information. There is also a significant effort to apply systems-level modeling to organize such data sets, bringing this field in close alignment with computational biology. Increasingly, synthetic biological concepts are being applied in the realm of therapeutics, including “designer” immune cells for the treatment of cancer.
Faculty working in systems & synthetic biology:
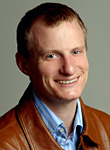
Associate Professor, Bioengineering
Anderson Lab develops new applications and tools for the Synthetic Biology community. Our goal is to create a computationally-driven platform for the design of genetic organisms that minimizes the uncertainties and errors of such projects. Our platform is built around a computational method for encapsulating the function of biomolecules based on precise chemical models. Our platform aggregates data from various sources, then develops synthesis and verification tools to automatically design engineered organisms with new functions, demonstrated with bacterium that produces acetaminophen.
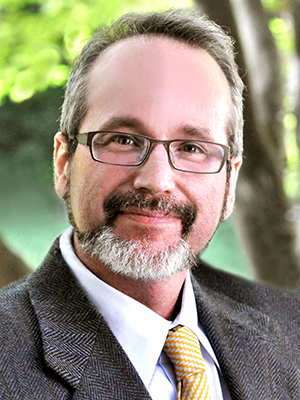
Dean A. Richard Newton Memorial Professor, Bioengineering; Senior Faculty Scientist, Environmental Genomics and Systems Biology Division, Lawrence Berkeley National Laboratory; Director, Center for the Utilization of Bioengineering in Space; CEO/CSO, DOE Systems Biology Knowledgebase PI and Co-Director, ENIGMA SFA
The Arkin Lab focus is how microbes transform, clean, and improve soils, soils that are currently degrading due to climate change, pollution, and poor water use. Near close-loops, low-energy, low-input biomanufacturing programs for food, pharmaceuticals, and building materials at “small village” scale, which are initially designed for a deep-space crewed Mars mission but have applications here on Earth for supporting sustainable agriculture. Another interest is to develop engineering approaches for microbiomes so we can control communities of microbes that drive the earth’s mineral cycles, support our plants and efficiency and stress responses, and impact the health and food-efficiency of a good many living creatures including ourselves.
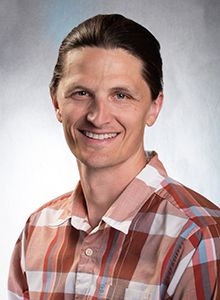
Assistant Professor, Bioengineering
The Clark Lab develops microfluidic and molecular methods for the high throughput analysis of single cells. We use these techniques to study HIV latency in CD4 T cells and profile cellular interactions during central nervous system inflammation.
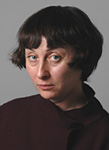
Professor, Bioengineering
Our work has been focused on establishing new paradigms in multi-tissue stem cell aging, rejuvenation and regulation by conserved morphogenic signaling pathways. One of our goals is to define pharmacology for enhancing maintenance and repair of adult tissues in vivo. The spearheaded by us heterochronic parabiosis and blood apheresis studies have established that the process of aging is reversible through modulation of circulatory milieu. Our synthetic biology method of choice focuses on bio-orthogonal non-canonical amino acid tagging (BONCAT) and subsequent identification of age-imposed and disease-causal changes in mammalian proteomes in vivo. Our drug delivery reg medicine projects focus on CRISPR/Cas9 based therapeutics for more effective and safer gene editing.

Lester John and Lynne Dewar Lloyd Distinguished Professor, Bioengineering
The Dueber Lab develops strategies for introducing designable, modular control over living cells. We are particularly interested in generating technologies for improving engineered metabolic pathway efficiency and directing flux. Our projects have applications in the development of biofuels, specialty chemicals, and environmentally friendly processes.
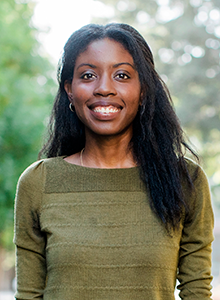
Assistant Professor
The Guthrie lab investigates the principles that govern microbial metabolism and signaling in the context of kidney homeostasis and disease using mass spectrometry, chemoinformatics, and molecular biology approaches.
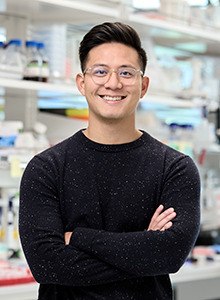
The Hsu Lab aims to understand and manipulate the genetic circuits that control brain and immune cell function to improve human health. We explore the rich biological diversity of nature to create new molecular technologies, perturb complex cellular processes at scale, and develop next-generation gene and cell therapies. To do this, our group draws from a palette of experimental and computational techniques including CRISPR-Cas systems, single cell genomics, engineered viruses, brain organoids, and pooled genetic screens.
Current interests include 1) inventing novel approaches for editing the postmitotic genome, 2) developing engineered vehicles for therapeutic macromolecule delivery, and 3) leveraging library screens and brain organoids to interrogate human neuroscience at scale.
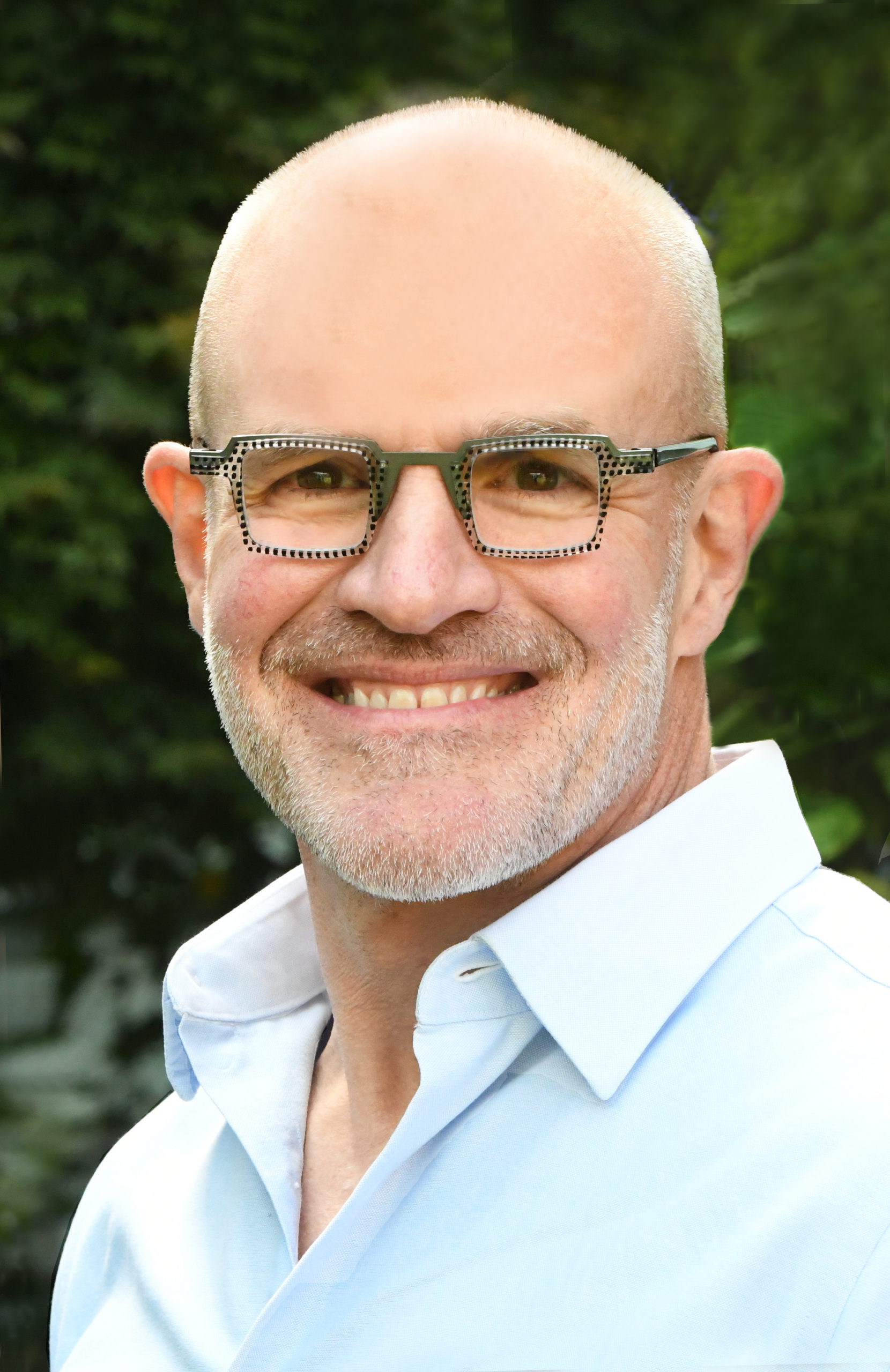
Professor, Bioengineering, Philomathia Professor of Alternative Energy at the University of California, Berkeley in the Departments of Bioengineering and Chemical and Biomolecular Engineering, senior faculty scientist at Lawrence Berkeley National Laboratory, and Chief Executive Officer of the Joint BioEnergy Institute (JBEI)
Metabolic engineering, environmental biotechnology, and biochemical engineering.

Chancellor’s Professor, Bioengineering & Chemical and Biomolecular Engineering Director, California Institute for Quantitative Biosciences (QB3) at UC Berkeley Professor in Residence, Bioengineering and Therapeutic Sciences, UCSF Faculty Scientist, Biological Systems and Engineering, LBNL
Our lab seeks to understand and engineer mechanical and other biophysical communication between cells and materials. In addition to investigating fundamental aspects of this problem with a variety of micro/nanoscale technologies, we are especially interested in discovering how this signaling regulates tumor and stem cell biology in the central nervous system. Recent directions have included: (1) Engineering new tissue-mimetic culture platforms for biophysical studies, molecular analysis, and screening; (2) Exploring mechanobiological signaling systems as targets for limiting the invasion of brain tumors and enhancing stem cell neurogenesis; and (3) Creating new biomaterials inspired by cellular structural networks.
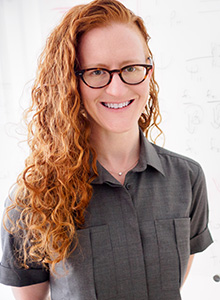
A single genome produces the huge diversity of cells and tissues needed to make a human by regulating gene expression to turn on and off the right genes at the right times. The final, post-transcriptional steps of gene expression — RNA processing and translation — are essential to the proper outcome. Our goal is to understand how these layers of regulation are encoded in gene sequences and how disruptions to this regulation can cause disease. Our research uses machine learning and other computational methods, coupled with high-throughput experiments, to understand how post-transcriptional regulation leads to robust and flexible control of gene expression.
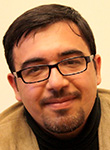
Professor, Bioengineering Professor, Mechanical Engineering Faculty Scientist, Lawrence Berkeley National Lab
Molecular and Multiscale Biomechanics; Bioinformatics and Computational Biology; Statistical Machine Learning; Computational Precision Health; Microbiome; Personalized Medicine
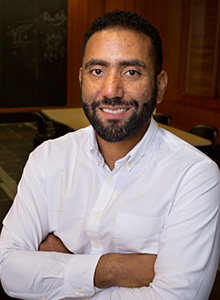
The Streets lab is interested in applying lessons from mathematics, physics, and engineering, to invent tools that help us dissect and quantify complex biological systems. Our goal is to uncover laws that govern the interactions of molecules inside the cell and the interactions between cells in a tissue or organism, by making precision measurements on single cells. In pursuit of this goal, we exploit three core technologies; microfluidics, microscopy, and genomics.
Accessibility

- English Language Programs
- Postdoctoral Affairs
- Training Grant Support
- Request Information
THE GRADUATE SCHOOL
- Academic Programs
- Clusters and Certificates
- Synthetic Biology
Synthetic Biology (Certificate)
- Certificate Requirements
Synthetic biology aims to understand and harness the rules of life toward engineering goals that benefit society. From molecules, to cells, to organisms, to biological communities and ecosystems, life all around us presents an enormous diversity of biological function that spans multiple spatiotemporal scales. These functions—from the abilities of cells to synthesize small molecules, remediate environmental contaminants, build, and maintain ecosystems, and differentiate to protect our immune systems—have great potential to become components of sustainable solutions for meeting pressing global challenges.
The Synthetic Biology TGS certificate curriculum emphasizes the physical and chemical principles of biological function in the context of building biological systems to understand the rules of life. The Synthetic Biology TGS certificate includes two introduction courses that teach the principles of synthetic biology and uses real-world case studies—recent landmark thrusts to build biological solutions to compelling societal challenges—to deconstruct biological phenomena along biological scales: molecular, circuit/network, cell/cell-free system, communities, and ecosystems. The curriculum then takes three additional topical courses categorized along the scales framework that delve deeper into the principles and tools used to engineer biological systems on a particular scale. A course in responsible conduct of research completes the training for the graduate certificate in synthetic biology.
A graduate certificate in synthetic biology will provide official recognition that students have received a multifaceted education in synthetic biology through Northwestern’s unique approach to synthetic biology training and prepare graduates to enter the biotechnology workforce.
See Synthetic Biology Certificate Requirements for specific courses and procedures needed to complete this program.
How to apply
Enrolled PhD and Master's students in The Graduate School may pursue this certificate with the permission of their program. In order to petition to have a Graduate Certificate awarded and appear on the transcript, students must submit the Application for a Graduate Certificate once all Graduate Certificate requirements have been completed, but no later than the time that the student files for graduation (in the final quarter of study).
Who to contact
Please contact the program director, listed below, with questions about this program.
- Program Director: Julius Lucks
- Email: [email protected]
You may also contact the Center for Synthetic Biology’s NSF NRT program for further questions at [email protected] .
The following requirements are in addition to, or further elaborate upon, those requirements outlined in The Graduate School Policy Guide .
In addition to meeting the PhD/MS requirements of their chosen departments, s tudents will be required to complete the coursework described below :
- CHEM ENG 376 – Principles of Synthetic Biology
- CHEM ENG 395 – Deconstructing Synthetic Biology
- IBiS 423 (Ethics in Biological Research)
- GEN ENG 519 (Responsible Conduct of Research)
- CHEM 519 (Responsible Conduct of Research Training)
- DGP 494-1 (Colloquium on Integrity in Biomedical Research)
- Three elective courses chosen by students as described below.
Elective Courses
Elective courses are organized into scale areas and methods/skills courses that reinforce the scales framework for synthetic biology training. Each course provides rigorous training in the fundamentals of physics, chemistry, and biology needed to understand biological function at a particular scale and technical approaches that can be used to apply the concepts of synthetic biology to engineer and manipulate the functions at that scale.
Interfaces between scales will be emphasized by the requirement of students to choose three electives that cover at least two different categories below:
- Molecular scale courses cover the physical, chemical and mathematical principles required for understanding the molecular basis of life and its use in biotechnology. Appropriate topics for these courses include biophysics of molecular folding, free energy landscapes, kinetic molecular folding, charge screening, molecular interactions, RNA folding, protein folding, enzymology, and others. Courses that use these principles to teach concepts related to RNA and protein design and experimental strategies for RNA and protein engineering are encouraged.
- Network/circuit scale courses enable students to understand biological, mathematical, and biophysical principles underpinning the mechanisms that biological systems utilize to propagate information, coordinate physiological states, and implement control over those states. These courses cover topics such as genetic circuits, metabolism, dynamical systems, network theory and mechanisms for intracellular and intercellular signaling and communication.
- Cell/cell-free systems scale courses cover biophysical and chemical principles involved in engineering biological parts within living and cell-free systems. These courses can include topics such as cellular and cell-free enzymatic biosynthesis, the implementation of genetic circuits in cell and cell-free systems, transport phenomenon at the cellular scale, interactions between cells/tissues and biomaterials, techniques for the manipulation of systems at this scale, and the use of cell-free systems as platforms for discovery and diagnostics.
- Biological communities scale courses will cover the biological, biochemical and mathematical principles required for understanding the emergent behavior of cellular communities. These courses can include topics such as microbial ecology and metagenomics, prediction of emergent microbial community dynamics, interspecies metabolic interaction, tissue-scale phenomena such as tissue engineering, microbial ecology, and modeling of biological communities including agent-based models and nonlinear differential equation models.
- Societal scale courses will teach students the skills needed to quantitatively estimate the needs, market sizes and viability of synthetic biology technologies including frameworks of field trials, user testing, and stakeholder analysis. These courses can also be used to address topics such as bioethics related to synthetic biology.
- Methods/skills courses teach students technical approaches that are important for applying concepts learned in other courses to their research or future careers. These courses can cover both experimental and computational approaches.
For a full list of electives that satisfy these criteria please visit: https://syntheticbiology.northwestern.edu/education/the-graduate-school-certificate-in-synthetic-biology.html
- Publications
Systems Biology Home Columbia University Department of Systems Biology
Columbia university medical center, personalized gene delivery to the gut.
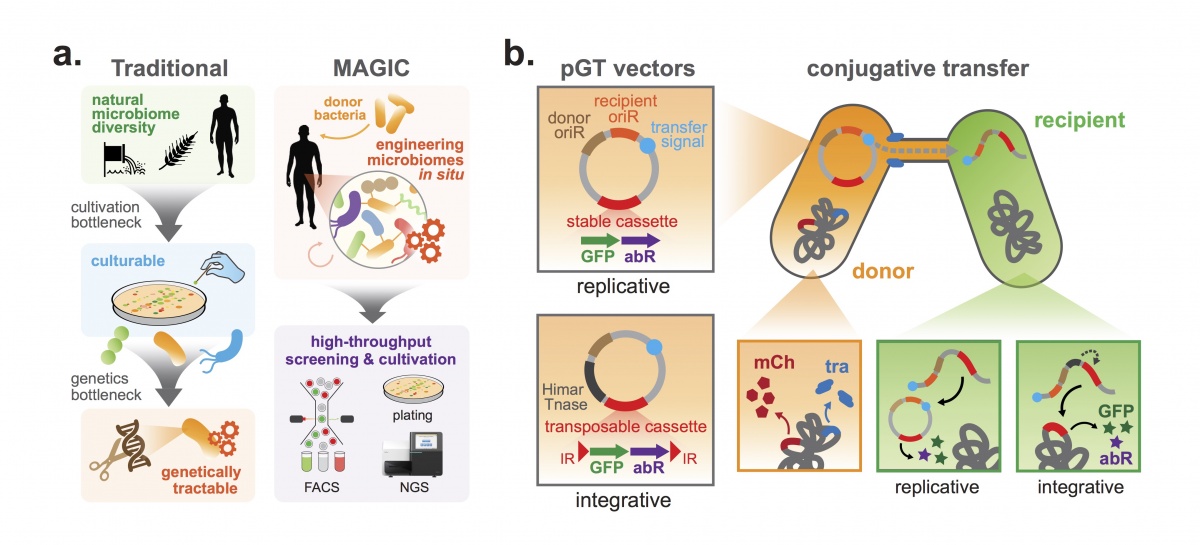
Illustrated here: (a) In contrast to traditional approaches to cultivate microbes first and then test for genetic accessibility, MAGIC harnesses horizontal gene transfer in the native environment to genetically modify bacteria in situ. (b) MAGIC implementation to transfer replicative or integrative pGT vectors from an engineered donor strain into amenable recipients in a complex microbiome. Replicative vectors feature a broad-host range origin of replication (oriR), while integrative vectors contain a transposable Himar cassette and transposase. The donor E. coli strain contains genomically integrated conjugative transfer genes (tra) and a mCherry gene. Transconjugant bacteria are detectable based on expression of an engineered payload that includes GFP and an antibiotic resistance gene (abr).
A team of researchers, led by Dr. Harris Wang of the Department of Systems Biology , has engineered bacteria to benefit and improve the overall health of our gut microbiome. In a proof-of-concept paper published in Nature Methods , Dr. Wang and his team demonstrate MAGIC, an innovative gene delivery system that ‘hacks’ the gut microbiome to perform any desired function, from harvesting energy from food and protecting against pathogen invasion to bolstering anti-inflammatory properties and regulating immune responses.
“The MAGIC system allows us to insert new gene functions directly into an existing microbiome without permanently altering the composition of the microbiome as a whole,” says Sway Chen , an MD/PhD student in the Wang lab and co-author of the study.
Designer Proteins Come with Built-In Safeguards
Protein engineering is a relatively young field that creates new proteins never seen before in nature. Today’s protein engineers usually create synthetic proteins by making small changes to the gene that encodes a naturally occurring protein. The variety of synthetic proteins range from stain-removing enzymes that have improved detergents to a long-acting insulin that’s used by millions of people with diabetes.
But two big unsolved challenges for protein engineers remain: The gene encoding the synthetic protein needs to be contained to prevent escape into other organisms and the gene needs to resist mutating over time so the protein doesn’t lose its function.
By merging two genes into a single DNA sequence, Columbia University synthetic biologists have created a method that could prevent human-engineered proteins from spreading into the wild, as well as stabilize synthetic proteins so they don’t change over time. The work, recently published in Science , was developed by Harris Wang , PhD, assistant professor of systems biology , with graduate student, Tomasz Blazejewski and postdoctoral scientist, Hsing-I Ho , PhD.
In devising the method, the researchers were inspired by overlapping genes in viruses. When two different genes overlap, they occupy the same sequence of DNA. But the genes are read in different frames so that two different proteins are produced.
In overlapping genes, a random mutation in the sequence may not affect one gene, but it’s likely that it will harm the second gene.
“Overlapping genes essentially lock in a specific DNA sequence, and we thought we could exploit this idea for synthetic biology ...Ten years ago, we didn’t have the technology that would make this possible,” says Dr. Wang. “We didn’t have enough sequences in the database to make informed predictions and we didn’t have a way to synthesize long DNA sequences for testing our predictions.”
Harris Wang’s Lab Receives Funding from the Gates Foundation to Study Childhood Mortality in Sub-Saharan Africa
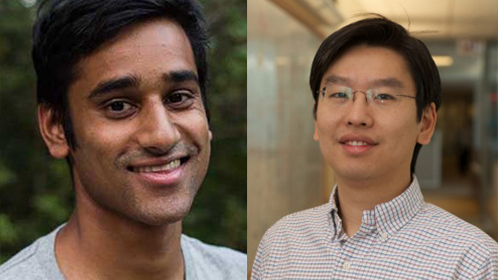
Dr. Harris Wang , PhD, and systems biology graduate student, Ravi Sheth , have been awarded a new grant from the Bill and Melinda Gates Foundation to help advance a global health project aimed at reducing childhood mortality in sub-Saharan Africa. The project incorporates Dr. Wang’s innovative microbiome research techniques and applies them to study the antibiotic, Azithromycin, towards understanding its role as an intervention for improving childhood survival rates in rural low-income, low-resource settings.
The study supported by the Gates grant expands on breakthrough research conducted in the MORDOR study , a cluster-randomized trial in which communities in Malawi, Niger and Tanzania were assigned to four twice-yearly mass distributions of either oral Azithromycin or placebo. Children, as young as 12 months of age, participated, and results indicated that the all-cause mortality rate was significantly lower for communities receiving the antibiotic versus placebo.
“This is an extremely exciting and, in many ways, very surprising result for such an underserved population,” says Sheth, who is a fourth-year PhD student in the systems biology track at Columbia University Irving Medical Center (CUIMC) . “Now it is crucial to understand how Azithromycin is acting to increase survival in such a profound way – to aid scale-up of the intervention and to help optimize the treatment regime and minimize any unintended consequences.”
The researchers will focus on developing a mechanistic understanding of how Azithromycin reshapes the gut microbiome, and how this altered microbiome state affects the host. The effect of the antibiotic will be studied over space and time to understand the perturbation to the gut ecosystem and resulting community re-configuration and re-assembly, and this information will be utilized to predict and test optimal dosing strategies.
New Resource for Controlling Gene Expression in Bacteria
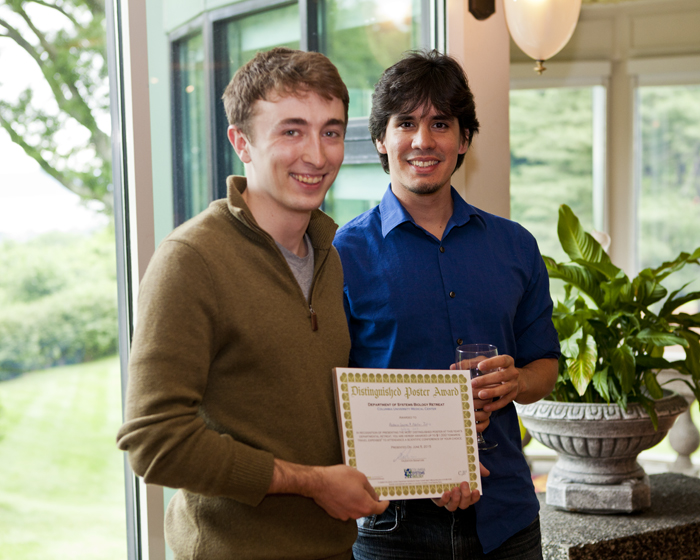
Advances in synthetic biology have already spurred innovation in the areas of drug development, chemical production and health diagnostics. To help push the field even further, and potentially at a more rapid pace, a new, comprehensive resource devised by Columbia University investigators will help synthetic biologists better engineer designs for complex biological systems.
A team of researchers, led by Harris Wang, PhD, assistant professor of systems biology and of pathology and cell biology, report the characterization and analysis of thousands of bacterial regulatory elements in different species of bacteria. The paper , published March 19, appears in Nature Methods .
Synthetic biology employs well-characterized genetic parts to assemble gene circuits with specific functions, such as producing chemicals or sensing the environment. The toolbox of genetic parts to make functioning genetic circuits, however, has been limiting. A key shortfall is the availability of precisely measured regulatory sequences-segments of DNA responsible for dialing up or dialing down the expression of proteins within an organism. For many commercially useful bacteria, tuning gene expression has been challenging because of a lack of reliable regulatory sequences.
"Synthetic biology is now at a precipice where we are not just demonstrating proof-of-concept in the laboratory but we're moving toward real-world applications," says Nathan Johns , lead coauthor of the paper, a member of the Wang Lab and a graduate student in the Department of Systems Biology at Columbia. "To facilitate this, having a wide array of useful genetic components and measurement techniques-in our case, regulatory sequences-are extremely helpful."
Harris Wang Named 2018 Schaefer Scholar

Harris Wang, PhD, assistant professor of systems biology, has been named a 2018 Schaefer Research Scholar for his novel approach to explore the role that bacteria cells in our gastrointestinal tract play on the efficacy of drug therapies.
Dr. Wang, who has a joint appointment in the Department of Pathology and Cell Biology, develops new tools and platforms to determine how genomes in microbial populations form, maintain themselves, and change over time, across many environments. His goal is to use synthetic biology approaches to engineer ecologies of microbial populations, such as those found in the gut and elsewhere in the human body, in ways that could improve human health.
The project that won the support of the Schaefer Scholars Research program centers on developing a platform approach to systematically determine new mechanisms by which specific members of the human microbiome metabolize and alter drugs and pharmaceuticals. Dr. Wang and his group intend to evaluate the impact of the microbiome on drug efficacies using cellular and animal models, focusing on the gut microbiome—an important and underexplored area of research.
“There have been studies that suggest a key link between microbes and their role altering the efficacy of drug treatments,” says Dr. Wang, “but this area of research is unchartered territory, and there is more knowledge to be gained by pinpointing how a person’s microbiome could metabolize specific therapeutics by inactivation, degradation, or alteration of its chemical structures. The large-scale data generated from our project could improve drug prescriptions and clinical trials by reducing failures and classifying patients based on otherwise unknown yet important microbiome-drug interactions.”
Harris Wang Named Recipient of Presidential Early Career Award for Scientists and Engineers
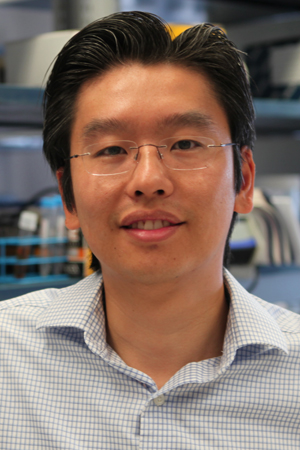
Harris Wang has been named a recipient of the prestigious Presidential Early Career Award for Scientists and Engineers (PECASE). Dr. Wang is among 102 researchers recognized today by President Barack Obama as the newest recipients of this honor.
The PECASE is considered the United States’ highest award for young scientists and engineers, conferred annually at the White House at the recommendation of participating federal agencies. The award celebrates young researchers at the beginning of their independent research careers who show exceptional promise to lead at the frontiers of twenty-first century science and technology.
A Grand Challenge for Genome Engineering: Virginia Cornish and Harris Wang on the GP-write Project
Department of Systems Biology bioengineer Harris Wang describes the goals of the Human Genome Project - Write (HGP-write), an international initiative to develop new technologies for synthesizing very large genomes from scratch.
In June 2016, a consortium of synthetic biologists, industry leaders, ethicists, and others published a proposal in Science calling for a coordinated effort to synthesize large genomes, including a complete human genome in cell lines. The organizers of the project, called GP-write (for work in model organisms and plants) or sometimes HGP-write (for work in human cell lines), envision it as a successor to the Human Genome Project (retroactively termed HGP-read), which 25 years ago promoted rapid advances in DNA sequencing technology. As the ability to read the genome became more efficient and less expensive, it in turn enabled a revolution in how we study biology and attempt to improve human health. Now, by coordinating the development of new technologies for writing DNA on a whole-genome scale, GP-write aims to have a similarly transformative impact.
Among the paper’s authors were Virginia Cornish and Harris Wang , two members of the Columbia University Department of Systems Biology whose contributions to the field of engineering biology have in part made the idea of writing large-scale DNA sequences imaginable. We spoke with them to learn more about what GP-write hopes to accomplish, its potential benefits, and how the effort is evolving.
Graduate Students Invent Technique for Reprogramming Translation
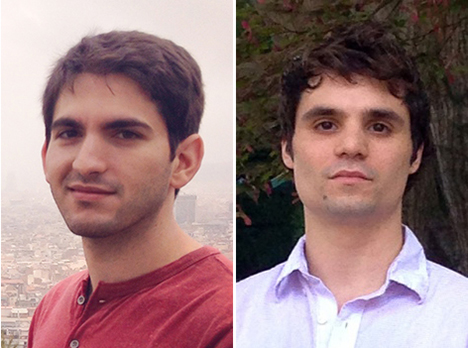
The ribosome is a reliable machine in the cell, precisely translating the nucleotide code carried by messenger RNAs (mRNAs) into the polypeptide chains that form proteins. But although the ribosome typically reads this code with uncanny accuracy, translation has some unusual quirks. One is a phenomenon called -1 programmed ribosomal frameshifting (-1 PRF), in which the ribosome begins reading an mRNA one nucleotide before it should. This hiccup bumps translation “out of frame,” creating a different sequence of three-nucleotide-long codons. In essence, -1 PRF thus gives a single gene the unexpected ability to code for two completely different proteins.
Recently Andrew Anzalone , an MD/PhD student in the laboratory of Virginia Cornish , set out to explore whether he could take advantage of -1 PRF to engineer cells capable of producing alternate proteins. Together with Sakellarios Zairis , another MD/PhD student in the Columbia University Department of Systems Biology , the two developed a pipeline for identifying RNA motifs capable of producing this effect, as well as a method for rationally designing -1 PRF “switches.” These switches, made up of carefully tuned strands of RNA bound to ligand-sensing aptamers, can react to the presence of a specific small molecule and reliably modulate the ratio in the production of two distinct proteins from a single mRNA. The technology, they anticipate, could offer a variety of exciting new applications for synthetic biology. A paper describing their approach and findings has been published in Nature Methods .
Columbia Launches Undergraduate Synthetic Biology Team
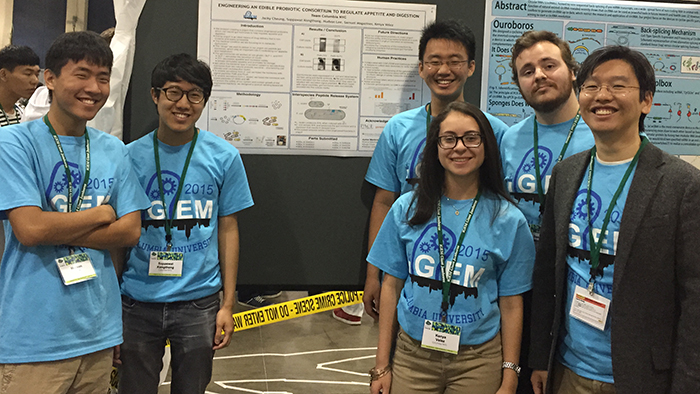
The Columbia University 2015 iGEM Team (l-r): Hudson Lee, Suppawat Kongthong, Jacky Cheung, Kenya Velez, Samuel Magaziner, and faculty moderator Harris Wang.
A team of undergraduate students based at Columbia University for the first time participated in this year’s International Genetically Engineered Machine Foundation (iGEM) competition . Supervised by Department of Systems Biology Assistant Professor Harris Wang , the team spent this past summer developing a project that used synthetic biology methods to engineer an edible, probiotic consortium of bacteria that could regulate hunger and digestion. In September they presented their results at the iGEM Giant Jamboree in Boston, MA, where they received a silver medal for their efforts. (For more informtion about their project, see the Columbia iGEM Team website .)
“I think it’s fantastic that this ambitious group of undergraduates worked so hard to represent Columbia University on this international stage,” says Dr. Wang. “Columbia has one of the great undergraduate colleges, and now that we have a critical mass of interested students and faculty laboratories with expertise in synthetic biology, we think iGEM offers a valuable opportunity to compete with and learn from teams at other leading institutions.”
Harris Wang Named Office of Naval Research Young Investigator
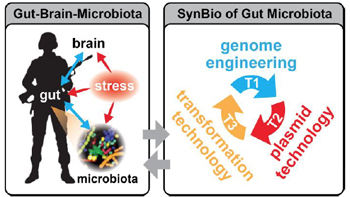
Harris Wang , an assistant professor in the Columbia University Department of Systems Biology, has been selected for the Office of Naval Research 2015 Young Investigators Program. This highly selective program promotes the development of early-career academic scientists whose research shows exceptional promise and creativity. With the support of this award, Dr. Wang will extend his research in the field of synthetic biology to develop new technologies for engineering the gut microbiome, the ecosystem of bacteria that inhabit the human digestive system. These new methods, Wang anticipates, could provide new ways of designing communities of different microbial species and ultimately modulating interactions between the gut, the immune system, and the brain.
Functional Metagenomics Enables First Study of Bacterial Fitness in the Gut Microbiome
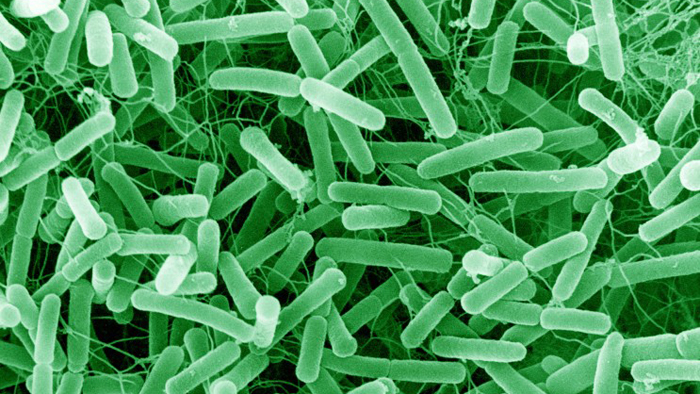
Photo by David Gregory and Debbie Marshall, Wellcome Images.
Recent deep sequencing studies are providing an increasingly detailed picture of the genetic composition of the human microbiome, the diverse collection of bacterial species that inhabit the gut. At the same time, however, little is known about the dynamics of these colonies, particularly why certain microbial strains outcompete others in the same environment. In a new paper published in the journal Molecular Systems Biology , Department of Systems Biology Assistant Professor Harris Wang , in collaboration with Georg Gerber and researchers at Harvard University, report on their development of the first method for using functional metagenomics to identify genes within commensal bacterial genomes that give them an evolutionary fitness advantage.
Harris Wang Named Winner of Sloan Research Fellowship
Harris Wang , an assistant professor in the Columbia University Department of Systems Biology and Department of Pathology and Cell Biology, has been selected to receive a 2015 Alfred P. Sloan Foundation Research Fellowship in computational and evolutionary molecular biology. This two-year, $50,000 grant will support work that combines methods from synthetic biology and computational biology to study how horizontal gene transfer contributes to microbial evolution.
Since 1955, the Sloan Research Fellowship program has supported outstanding early-career scientists in recognition of their achievements and their potential to make important contribution to their fields. This year’s fellows included 126 investigators, with 12 awardees in the field of computational and evolutionary molecular biology. Other disciplines represented in the awards include chemistry, computer science, economics, mathematics, neuroscience, ocean sciences, and physics.
New Directions in Genome Engineering: An Interview with Harris Wang
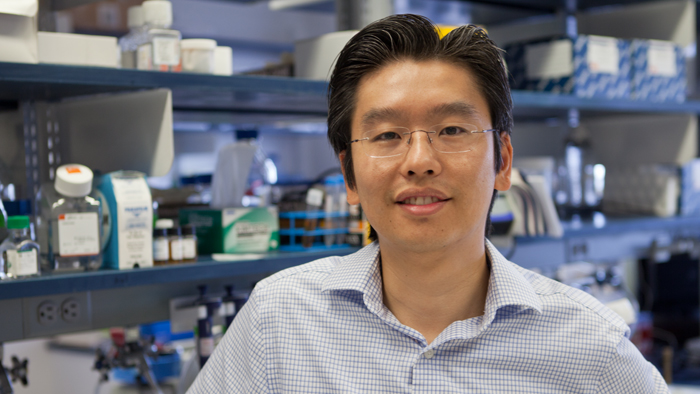
As a graduate student in George Church’s lab at Harvard University, Harris Wang developed MAGE, a revolutionary tool for the field of synthetic biology that made it possible to introduce genomic mutations into E. coli cells in a highly specific and targeted way. Now an Assistant Professor in the Columbia University Department of Systems Biology, Dr. Wang recently published a paper in ACS Synthetic Biology that introduces an important advance in the MAGE technology. The new technique, called (MO)-MAGE, uses microarrays to engineer pools of oligonucleotides that, once amplified and integrated into a genome, can generate thousands or even millions of highly controlled mutations simultaneously. This new method offers a cost-effective way for designing and producing large numbers of genomic variants and provides an efficient platform for experimentally exploring genome-wide landscapes of mutations in bacteria and optimizing the organisms’ biochemical capabilities.
In the following interview, Dr. Wang explains the origins of the new technology, and discusses what he sees as the remarkable potential it holds for both basic biological research and industrial applications of synthetic biology.
How are MAGE and (MO)-MAGE different from more traditional methods in genome engineering?
In traditional genome engineering, researchers would induce genome perturbations randomly. For example, you might use ultraviolet radiation or a mutagen to generate mutations and then do a selection experiment to compare and isolate cells with different genotypes based on how they respond to specific stimuli. The problem with this approach, though, is that you have no way to control what mutations occur, even if you know the mutation you are interested in investigating.
(MO)-MAGE offers a cost-effective and efficient way to simultaneously mutate large numbers of genes in a targeted way.
Synthetic and Systems Biology: Reinventing the Code of Life
A panel at the Helix Center, titled "Synthetic and Systems Biology: Reinventing the Code of Life included Columbia University professors Saeed Tavazoie and Andrea Califano, as well as Michael Hecht (Professor of Chemistry, Princeton University), Mark Fishman (President, Novartis Institutes for BioMedical Research), Christopher Mason (Assistant Professor of Physiology and Biophysics, Institute for Computational Biology, Weill Cornell Medical College), and Michael Waldholz (Medical Science Writer and Media Consultant).
Advances in genomics and the development of new technologies over the past decade have given biologists the ability to engineer DNA to perform specific functions. This emerging science, called synthetic biology, holds great potential for a number of applications, and experiments have already been done to reprogram algae to produce biofuels, design bacteria that can sense and consume toxic substances, and use living cells to manufacture compounds that can be used as drugs.
Synthetic biology has emerged in parallel with systems biology, but in many ways the two sciences are closely intertwined. As systems biology improves our mechanistic understanding of how biology functions at the molecular level, synthetic biology is taking this knowledge to push biology in new directions, from synthesizing molecules using biology all the way to synthesizing new forms of biological life.
In a public roundtable discussion at the Helix Center in New York City, Columbia University Department of Systems Biology professors Saeed Tavazoie and Andrea Califano joined a panel of experts in discussing the intersection of systems and synthetic biology, and the role that these two disciplines will play in the development of the biological and biomedical sciences in the coming years.
- Columbia Genome Center
- Center for Computational Biology & Bioinformatics (C2B2)
- Center for Cancer Systems Therapeutics (CaST)
- Center for Topology of Cancer Evolution and Heterogeneity
- Cancer Target Discovery & Development Center (CTD2)
- International Serious Adverse Event Consortium (iSAEC)
Infrastructure
- Genome Sequencing and Analysis
- High-Throughput Screening
- Information Technology (DSBIT)
Education & Training
- Graduate Education
- Postdoctoral Training
About the Department

Columbia University | Columbia University Irving Medical Center © 2019 Columbia University Irving Medical Center
Columbia University Department of Systems Biology Irving Cancer Research Center 1130 St. Nicholas Avenue, New York, NY 10032 (212) 851-4673
APPLY NOW FOR FALL 2024 ADMISSIONS

Synthetic Biology
Rice University has over 20 Synthetic Biology research groups, which share the goal of overcoming central challenges in engineering biology. These labs are pioneering new tools, technologies, and theories to transform our ability to predictably design biological systems. This includes the development of programmable biological parts for constructing genetic circuits, resolving design rules for creating multicellular genetic programs that have complex temporal and spatial behaviors, and developing safe strategies to test and use synthetic systems in real, complex settings. These groups apply these advances to a range of synthetic biology applications—delivering biological solutions to improve health, enable sustainable practices, and yield bioelectronics technologies.
Theme I. Cell-Based Therapeutics
Our faculty are focused on elucidating the function of cells as natural sensors and repurposing them as components in smart living therapeutics. Leveraging unique research strengths at Rice and its adjacency to the Texas Medical Center, efforts are focused on immune diseases, neurological diseases, and cancers. Faculty also leverage these sensors for the noninvasive imaging and manipulation of tissues deep within the body to study physiology and disease. Finally, our faculty are developing new modalities for engineering the microbial communities that live upon the human body to maintain health, treat diseases, and prevent infections.
Theme II. Living Electronics
Our faculty are developing synthetic molecules and cells that can be used as components within digital devices. They are creating genetic programs that enable cells to convert chemical information in the environment into electrical information in real-time and developing strategies to read this information out using digital devices. By interfacing synthetic cells with built materials, they are also working to create seamless two-way electrical communication between cells and devices to enable novel sensing and actuating applications that support our health and sustainable practices in the environment.
Theme III. Sustainable Living Materials
Our faculty are working to program cells to produce materials that rival the structure and function of natural materials. These efforts seek to extend beyond traditional metabolic engineering and to establish the knowledge required to control the biological synthesis and patterning of proteins and cells from the micron to meter scales. Through synthetic biology, these efforts are working to yield sustainable replacements for existing materials, impossible materials with physical properties unrivaled in conventional materials, and living materials that can self-replicate and self-repair.
Theme IV. Programming Environmental Consortia
Our faculty are studying how to transfer genetic circuits into environmental consortia without the need for organismal domestication, program precise, micron-scale sensing and actuation within cells of natural, structured microbial consortia, program control over cell-cell and cell-material interaction networks in heterogeneous environments, and achieve effective biocontainment within complex geological and built environments of relevance to future synthetic biology applications. These efforts all work to discover the underlying design principles by integrating experimental and computational approaches.
Training Faculty
- Caroline Ajo-Franklin
- Pedro Alvarez
- Caleb Bashor
- Matthew Bennett
- James Chappell
- Mingjie Dai
- Marcos de Moraes
- Jimmy Gollihar
- Isaac Hilton
- Theresa Loveless
- Carrie Masiello
- Hans Renata
- Laura Segatori
- Yousif Shamoo
- Joff Silberg
- Lauren Stadler
- Jerzy Szablowski
- Omid Veiseh
Helpful Links
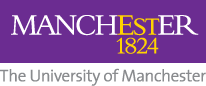
Alternatively, use our A–Z index
Attend an open day
Discover more about postgraduate research
PhD Synthetic and Systems Biology / Overview
Year of entry: 2024
- View full page
The standard academic entry requirement for this PhD is an upper second-class (2:1) honours degree in a discipline directly relevant to the PhD (or international equivalent) OR any upper-second class (2:1) honours degree and a Master’s degree at merit in a discipline directly relevant to the PhD (or international equivalent).
Other combinations of qualifications and research or work experience may also be considered. Please contact the admissions team to check.
Full entry requirements
Apply online
In your application you'll need to include:
- The name of this programme
- Your research project title (i.e. the advertised project name or proposed project name)
- Your supervisor's name
- If you already have funding or you wish to be considered for any of the available funding
- Details of your previous study
- Names and contact details of your two referees
Before applying we also recommend that you read the Advice to Applicants section.
Find out how this programme aligns to the UN Sustainable Development Goals , including learning which relates to:
Goal 3: Good health and well-being
Goal 11: sustainable cities and communities, goal 12: responsible consumption and production, goal 15: life on land, programme options, programme overview.
- Embrace the challenge of living abroad in one of the world's most famous cities.
- Form part of a talented cohort seeking to address sustainable development challenges through research in bio-economy solutions, and choose from a range of projects spanning synthetic and systems biology.
- Develop a global perspective and international fluency to benefit your future development, opening the door to exciting new job opportunities.
The programme fee will vary depending on the cost of running the project. Fees quoted are fully inclusive and, therefore, you will not be required to pay any additional bench fees or administration costs.
All fees for entry will be subject to yearly review and incremental rises per annum are also likely over the duration of the course for Home students (fees are typically fixed for International students, for the course duration at the year of entry). For general fees information please visit the postgraduate fees page .
Always contact the Admissions team if you are unsure which fees apply to your project.
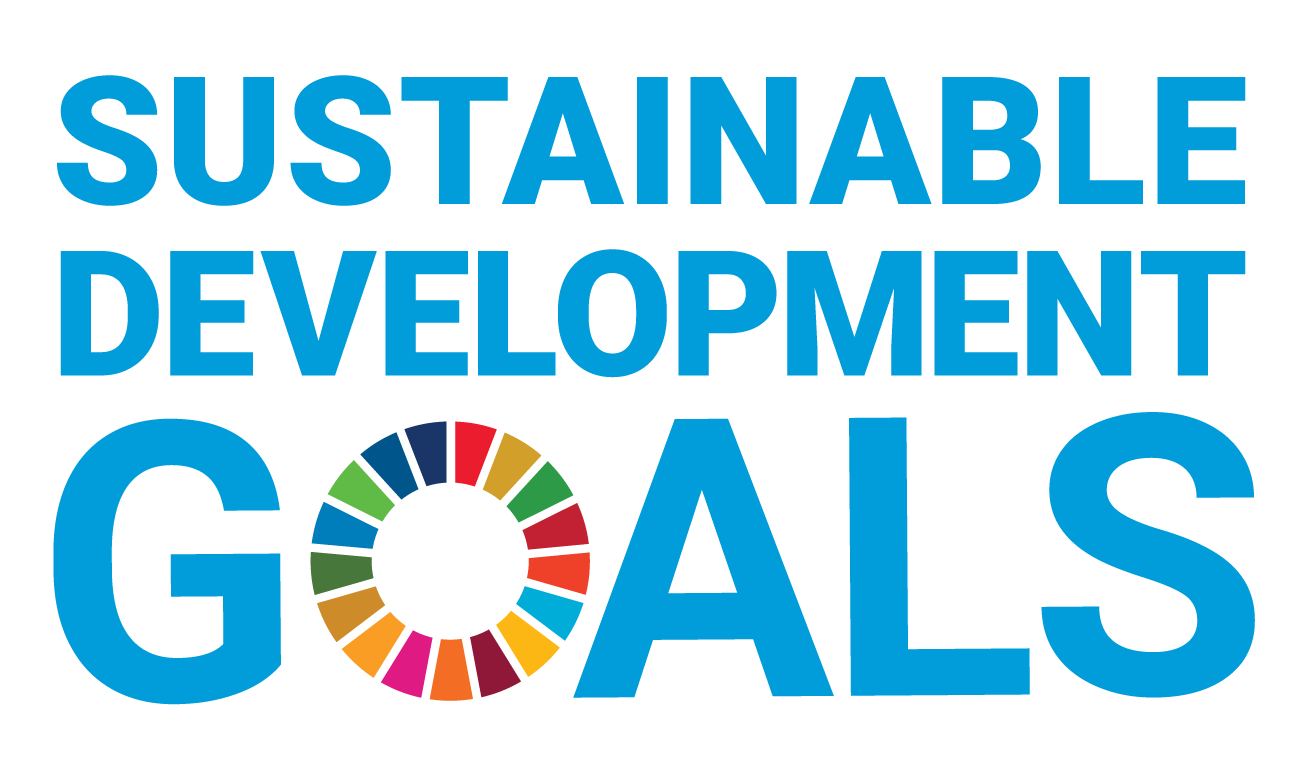
UN Sustainable Development Goals
The 17 United Nations Sustainable Development Goals (SDGs) are the world's call to action on the most pressing challenges facing humanity. At The University of Manchester, we address the SDGs through our research and particularly in partnership with our students.
Led by our innovative research, our teaching ensures that all our graduates are empowered, inspired and equipped to address the key socio-political and environmental challenges facing the world.
To illustrate how our teaching will empower you as a change maker, we've highlighted the key SDGs that our programmes address.
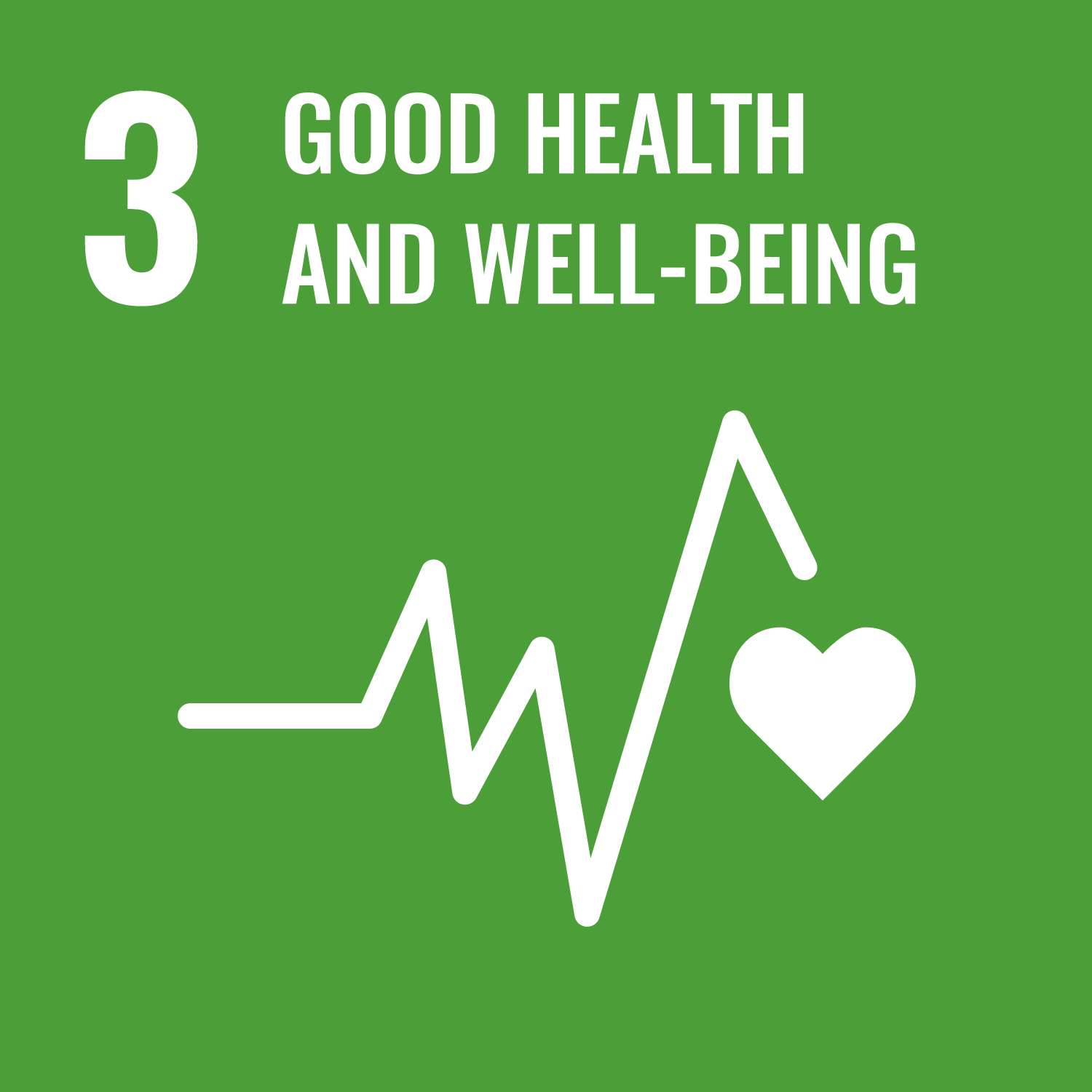
Ensure healthy lives and promote well-being for all at all ages
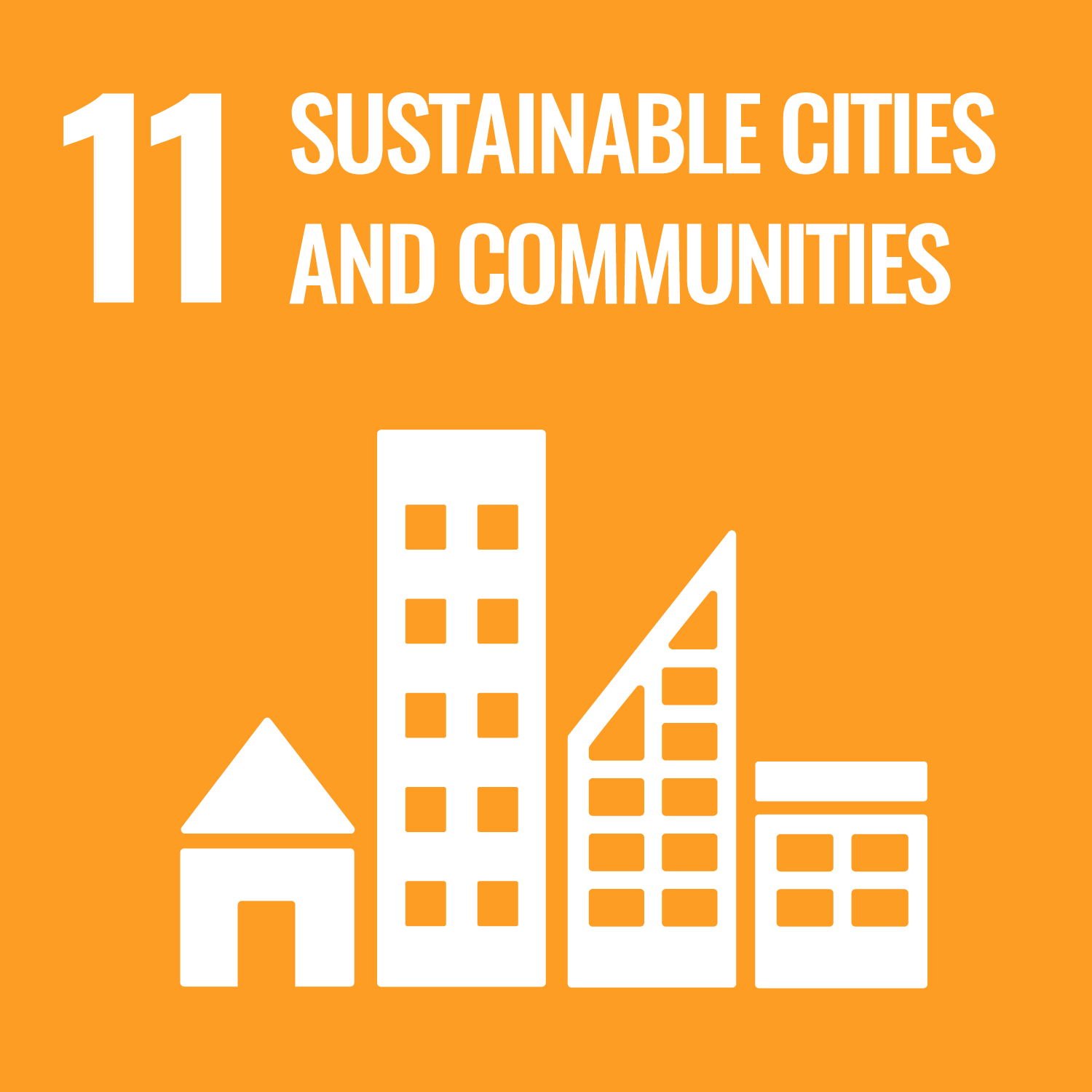
Make cities and human settlements inclusive, safe, resilient and sustainable
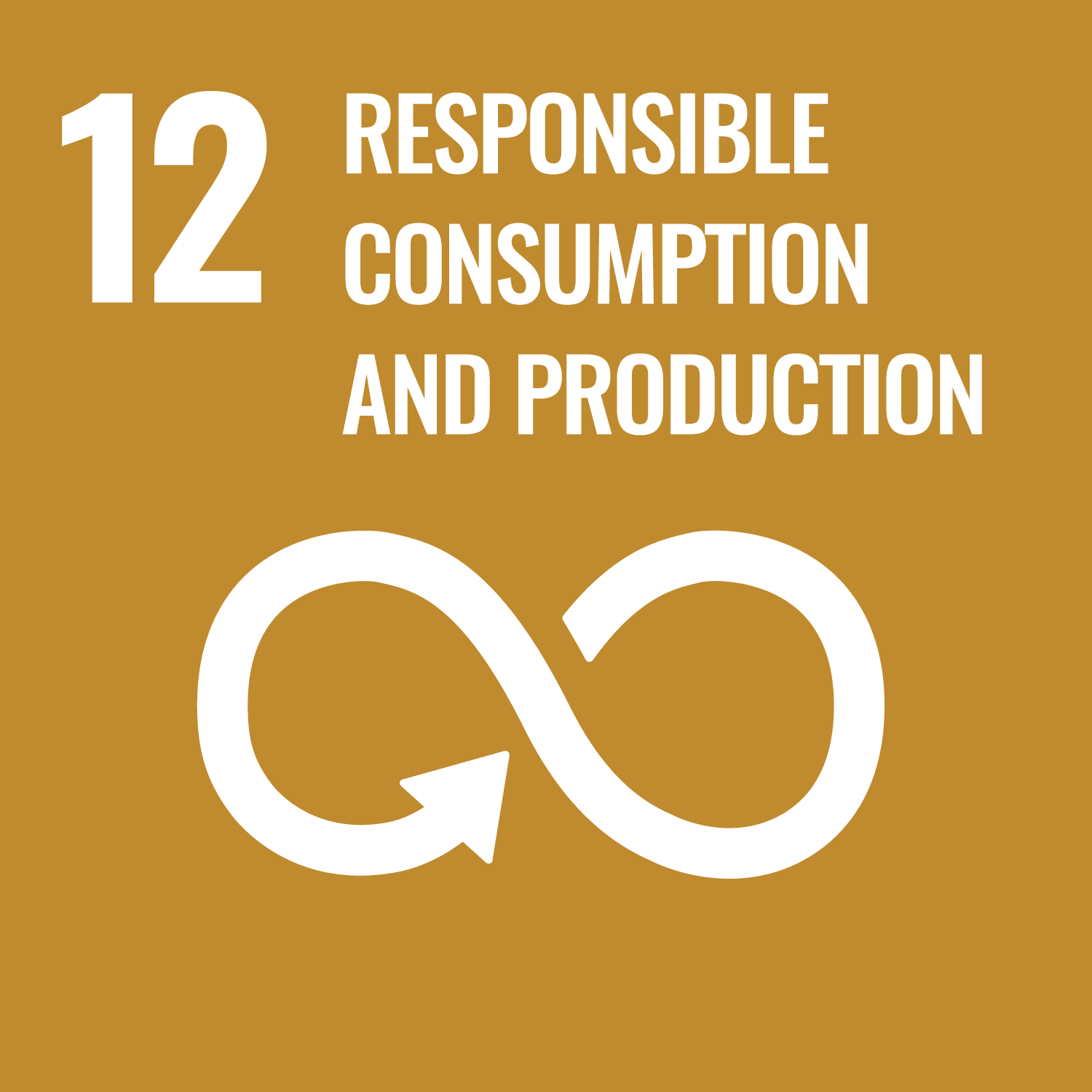
Ensure sustainable consumption and production patterns
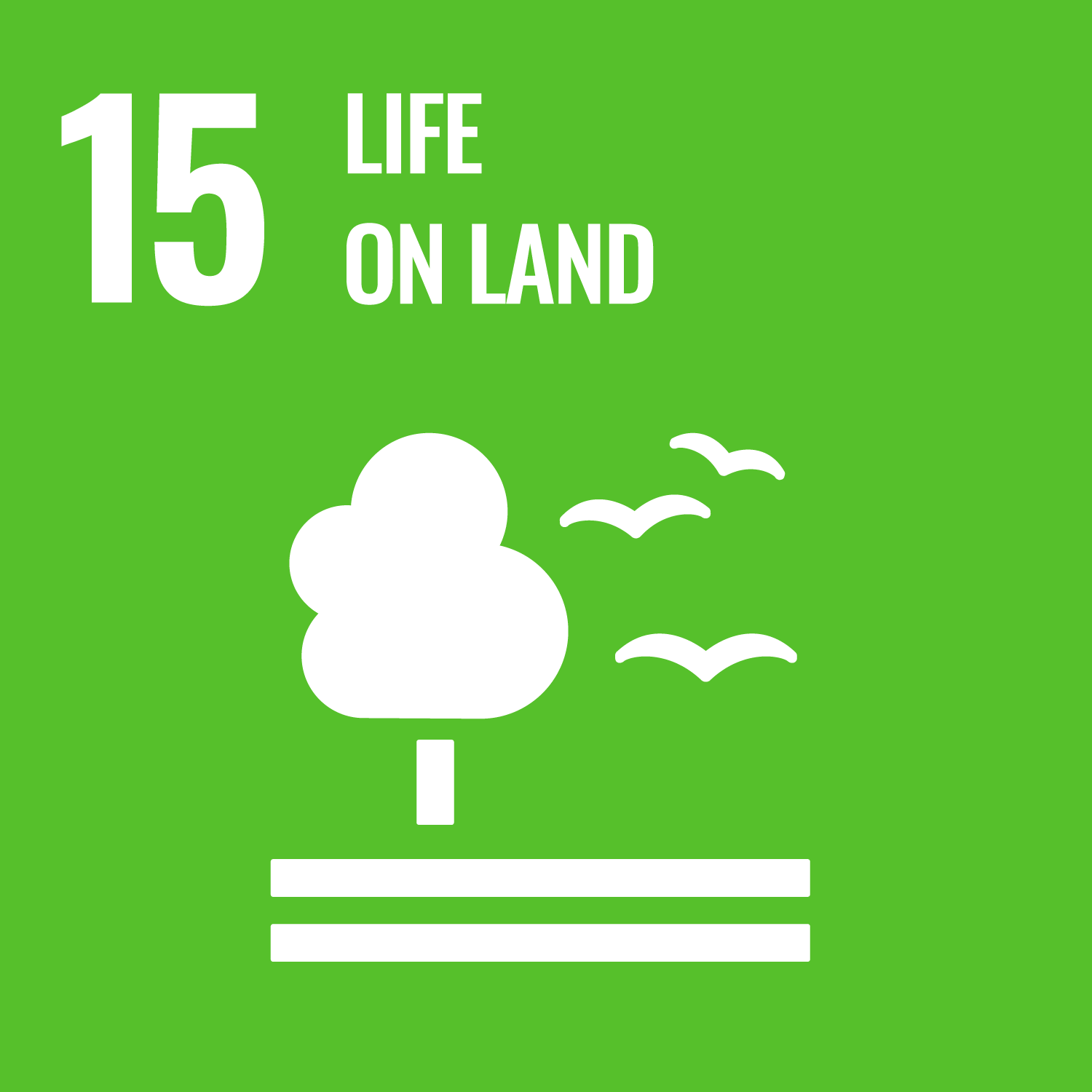
Protect, restore and promote sustainable use of terrestrial ecosystems, sustainably manage forests, combat desertification, and halt and reverse land degradation and halt biodiversity loss
Contact details
Our internationally-renowned expertise across the School of Natural Sciences informs research led teaching with strong collaboration across disciplines, unlocking new and exciting fields and translating science into reality. Our multidisciplinary learning and research activities advance the boundaries of science for the wider benefit of society, inspiring students to promote positive change through educating future leaders in the true fundamentals of science. Find out more about Science and Engineering at Manchester .
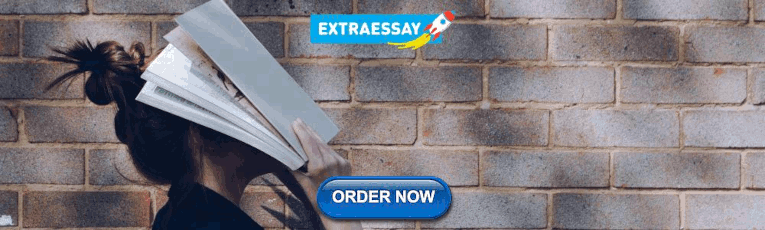
Programmes in related subject areas
Use the links below to view lists of programmes in related subject areas.
Regulated by the Office for Students
The University of Manchester is regulated by the Office for Students (OfS). The OfS aims to help students succeed in Higher Education by ensuring they receive excellent information and guidance, get high quality education that prepares them for the future and by protecting their interests. More information can be found at the OfS website .
You can find regulations and policies relating to student life at The University of Manchester, including our Degree Regulations and Complaints Procedure, on our regulations website .
Synthetic Biology 101
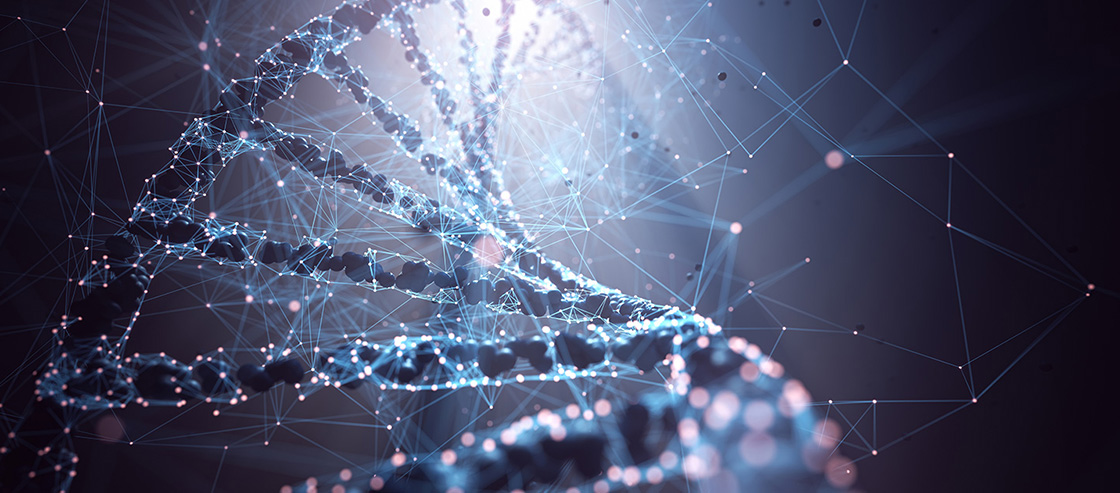
What is Synthetic Biology?
Synthetic biology is a discipline that uses biological components as building blocks in the design of new systems to solve crucial global challenges
By combining concepts across disciplines, researchers can build with biology in much the same way an engineer creates a high-tech device; but instead of the program running on a computer, it does so within a biological system. Advances in DNA sequencing, DNA synthesis, machine learning, and artificial intelligence have fueled synthetic biology’s growth, allowing for the development and scaling of new bioengineered solutions. These solutions can interface with every facet of our lives.
As estimated by the National Academies of Sciences, Engineering and Medicine, the bioeconomy is about five percent of US gross domestic product (one trillion dollars) and growing, compared to the approximate one percent that represents the U.S. semiconductor industry. Investments in the bioeconomy have given rise to billion-dollar companies that rely on synthetic biology for their products. Often, these companies are using biology to redesign traditional processes to produce familiar items in ways that are more environmentally sustainable.
Synthetic Biology Technology is All Around Us
Examples of synthetic biology appear everywhere
In agriculture, synthetic biologists have developed microbes that can make their own fertilizer, a development that could combat global hunger by increasing crop yield and aiding farmers in some of the world’s poorest regions. In the commodities industry, synthetic biologists have engineered a bacterium that can pull carbon dioxide and carbon monoxide out of the air, turning them into common chemicals like acetone and isopropanol—redirecting carbon emissions that are normally funneled into the atmosphere. In medicine, synthetic biology innovations such as mRNA vaccines are being used as tools to treat infectious diseases, helping public health experts and society stay a step ahead of the next pandemic and providing new approaches to teach our own immune systems to better detect and eliminate cancers.
The grocery store offers an even more well-known product of synthetic biology’s potential and reach. The Impossible Burger, a popular meat alternative, uses a lab-engineered non-meat-based heme molecule to help its product look and taste more like a traditional beef hamburger. The difference, according to an environmental analysis by independent auditor Quantis, is that production of one Impossible Burger patty uses 96 percent less land and 87 percent less water as compared to one beef patty, while also releasing 89 percent less carbon into the atmosphere. Such advances show just how significant synthetic biology’s benefits can be.
Investing in the Future
Synthetic biology is a national priority
The U.S. government took an early interest in synthetic biology research, with the National Science Foundation (NSF) providing a $37 million, 10-year grant in 2006 to fund a national Synthetic Biology Engineering Research Center. DARPA followed suit with multiple programs focused on synthetic biology. From 2008 to 2014, estimates suggest the government invested $820 million in synthetic biology research, spread across the NSF, the Department of Energy, the Department of Defense, and the National Institutes of Health (NIH).
The U.S. government underscored its intention to accelerate the growth of synthetic biology research when the White House issued an executive order in September 2022, launching a national biotechnology and biomanufacturing initiative that places synthetic biology as a centerpiece of our strategies for sustainability, competitiveness, and economic growth across all levels of government. The order’s impact has the potential to be far reaching including: significant investments to develop medicines and commodities, reduce waste, and advance sustainable farming, while also mitigating climate change impacts.
A Brief History
History in the Making
The roots of synthetic biology are planted with a landmark investigation by researchers François Jacob and Jacques Monod, whose study of E. coli led them to posit the existence of regulatory circuits that underpin the response of a cell to its environment.
January 2000
Two side-by-side reports are published by researchers at Boston University and Princeton University showing that biological protein parts can be engineered into genetic circuits to carry out designed functions.
January 2003
MIT launches an independent study course in which students develop biological devices to make cells blink; the following year the course evolves into a summer competition known as iGEM (International Genetically Engineered Machines). Northwestern would go on to launch its first iGEM program in 2010.
The first international conference for the field, Synthetic Biology 1.0, is held at MIT; future conferences in Switzerland and Hong Kong would help to establish the field’s global growth.
BioBricks Foundation is established as a nonprofit organization to catalog and standardize synthetic biology parts, providing a database of resources for scientists and researchers.
Funded by a 10-year grant from the National Science Foundation (NSF), the Synthetic Biology Engineering Research Center (SynBERC) is founded to advance research, innovation, training, and education in synthetic biology. In this time, SynBERC trains and provides early career support to future Northwestern synthetic biology faculty.
The World Health Organization approves the use of a semi-synthetic, non-plant-derived version of the antimalarial drug artemisinin as a low-cost alternative to treat malaria in developing nations—the first large-scale synthetic biology commercial endeavor.
The US Department of Energy prepares a report to Congress on synthetic biology, offering a comprehensive plan for federally supported research and development in support of energy and environmental goals.
Northwestern University launches its interdisciplinary Center for Synthetic Biology (CSB).
A group of leading scientists, including Northwestern CSB faculty, proposes a large-scale synthetic biology initiative, leading to the creation of the Human Genome Project-Write, which is focused on the synthesis of human genomes.
Northwestern University receives an NSF grant to fund a 10-week summer research experience in synthetic biology for undergraduate students, the first synthetic biology-focused, NSF-funded program of its kind.
September 2018
After SynBERC’s 10-year funding grant ends, Northwestern CSB faculty help found the Engineering Biology Research Consortium with an NSF award to support and sustain the impact of research, products, discoveries, and ideas from the synthetic biology community.
Northwestern University receives a $3 million NSF grant to support Synthesizing Biology Across Scales (SynBAS), a program focused on convergent synthetic biology training for graduate students, another first-of-its-kind synthetic biology-focused program.
September 2022
The United States launches a national biotechnology and biomanufacturing initiative.
PhD Position in Synthetic Biology
Job information, offer description.
Have you ever thought about the questions, “What is life?” and “Can we create life-like systems?”. Are you excited about the prospect of building artificial entities, using basic molecular components, that can mimic vital characteristics of living systems and have further biotechnological potential? Are you a creative thinker, eager to tackle an exciting synthetic biology project using state-of-the-art facilities in a multidisciplinary and a collaborative department? If so, this might be the perfect PhD position for you!
What will you do?
- develop and characterize phase-separating peptides aimed at achieving multi-compartmentalization within cell-mimicking vesicles;
- use and design on-chip microfluidic systems for vesicle production and controlled experimentation;
- perform fluorescence (confocal) microscopy and image analysis to study process dynamics;
- utilize physical chemistry techniques (scattering, AFM, spectroscopy, etc.) to complement the microscopy and lab-on-a-chip research;
- get involved in collaborative synthetic cell-related research with other consortium members.
Project description Living cells exhibit extensive sub-compartmentalization to regulate and communicate between thousands of interconnected biochemical reactions taking place within the cytoplasm. Along with well-known membrane-bound compartments, phase-separated membraneless organelles have been identified in recent years to play an equally important role in these processes. In this project, we will utilize coacervate-based strategy to form communicative membraneless compartments within cell-mimicking vesicles, with each of the compartments acting as distinct functional biochemical units. Being able to maintain distinct reaction hubs within vesicles will constitute an important step towards building intracellular communication networks. We will use a bioengineering approach, applying our microfluidic and protein engineering toolbox to design scalable multi-compartmentalization capable of organizing the intracellular reaction networks and pave a path towards complex synthetic cells.
This PhD position is part of a larger European consortium, SIGSYNCELL. This is a doctoral network funded by the European Commission via Marie-Sklodowska Curie Actions (MSCA), whose goal is training through research. It is a consortium that brings together a dozen European academic partners, in addition to private companies, coordinated by the CNRS in Bordeaux, France. SYGSYNCELL partners include CNRS (F), AMOLF (NL), University of Muenster (DE), University of Ljubjiana (SI), University of Granada (ES), Wageningen University (NL), Ruprecht-Karls-Universitaet Heidelberg (DE), and Imperial College London (UK).
Requirements
- you have an MSc degree (or equivalent) in biophysics, soft matter, nanotechnology, physical chemistry, or a related field;
- you have a strong affinity for interdisciplinary research and the capacity to dive into unexplored areas;
- prior experience in microfluidics, protein synthesis, image analysis, or programming and coding is a plus;
- you are capable of working independently but are also a good team player;
- you have good communication and presentation skills.
For this position your command of the English language is expected to be at C1 level . Sometimes it is necessary to submit an internationally recognised Certificate of Proficiency in the English Language. More information can be found here .
Mobility conditions
- MSCA networks require that applicants must not have studied/worked in the host country for more than 12 months in the 3 years immediately prior to the position’s start date;
- the individual scientific projects are planned within one of the partner Host Institutions and every Doctoral candidate will spend a part of their doctoral studies in another consortium laboratory (a maximum of 12 months of their 36-month PhD).
Additional Information
Wageningen University & Research offers excellent terms of employment . A few highlights from our Collective Labour Agreement include:
- partially paid parental leave;
- working hours that can be discussed and arranged so that they allow for the best possible work-life balance;
- the option to accrue additional compensation / holiday hours by working more, up to 40 hours per week;
- there is a strong focus on vitality and you can make use of the sports facilities available on campus for a small fee;
- a fixed December bonus of 8.3%;
- excellent pension scheme.
In addition to these first-rate employee benefits, you will receive a fully funded PhD position and you will be offered a course program tailored to your needs and the research team.
The gross salary for the first year is € 2.770 - per month rising to € 3.539,- in the fourth year in according to the Collective Labour Agreements for Dutch Universities (CAO-NU) (scale P). This is based on a full-time working week of 38 hours. We offer a temporary contract for 18 months which will be extended for the duration of the project if you perform well.
There are plenty of options for personal initiative in a learning environment, and we provide excellent training opportunities. We are offering a unique position in an international environment with a pleasant and open working atmosphere.
You are going to work at the greenest and most innovative campus in Holland, and at a university that has been chosen as the “ Best University ” in the Netherlands for the 19th consecutive time.
Coming from abroad Wageningen University & Research is the university and research centre for life sciences. The themes we deal with are relevant to everyone around the world and Wageningen, therefore, has a large international community and a lot to offer to international employees.
Our team of advisors on Dutch immigration procedures will help you with the visa application procedures for yourself and, if applicable, for your family.
Feeling welcome also has everything to do with being well informed. Wageningen University & Research's International Community page contains practical information about what we can do to support international employees and students coming to Wageningen. Furthermore, we can assist you with any additional advice and information about for example helping your partner to find a job, housing, or schooling.
Finally, certain categories of international staff may be eligible for a tax exemption on a part of their salary during the first five years in the Netherlands.
For more information about this position, please contact Dr. Siddharth Deshpande (assistant professor), by e-mail: [email protected] .
For more information about the procedure, please contact Rutger Voorrips (corporate recruiter), by e-mail: [email protected] .
Do you want to apply? You can apply directly using the apply button on the vacancy page on our website which will allow us to process your personal information with your approval. This vacancy will be listed up to and including Monday, 6 May 2024. We hope to schedule the first job interviews in June 2024.
Equal opportunities Wageningen University & Research (WUR) employs a large number of people with very different backgrounds and qualities, who inspire and motivate each other. We want every talent to feel at home in our organisation and be offered the same career opportunities. We therefore especially welcome applications from people who are underrepresented at WUR. A good example of how WUR deals with inclusiveness can be read on the page working at WUR with a functional impairment .
Work Location(s)
Where to apply.
Thank you for visiting nature.com. You are using a browser version with limited support for CSS. To obtain the best experience, we recommend you use a more up to date browser (or turn off compatibility mode in Internet Explorer). In the meantime, to ensure continued support, we are displaying the site without styles and JavaScript.
- View all journals
- Explore content
- About the journal
- Publish with us
- Sign up for alerts
- TECHNOLOGY FEATURE
- 01 April 2024
How synthetic biologists are building better biofactories
- Sara Reardon 0
Sara Reardon is a freelance journalist based in Bozeman, Montana.
You can also search for this author in PubMed Google Scholar
Synthetic small-molecule cofactors could reduce the cost of biomanufacturing. Credit: Alamy
Scientists have used microorganisms to produce beneficial chemicals for decades. By providing the microbes with enzymes and metabolic pathways, researchers can coax cells to churn out everything from food additives to biofuels.
One advantage of biomanufacturing is ecological: the processes are generally more environmentally friendly than are chemical manufacturing methods. But it’s expensive, mainly because cells won’t create something simply because researchers want them to. The enzymes needed to produce ethanol fuel from plant sugars, for example, must compete with the cell’s own metabolic enzymes for energy, and the yield can be poor.
Building complex products, such as pharmaceuticals, can involve numerous steps carried out by multiple enzymes. When the cell’s ability to develop is pitted against its ability to make a product it doesn’t need, “the cells are going to choose to grow every time”, says Brian Pfleger, a synthetic biologist at the University of Wisconsin–Madison.
Rather than attempting to win this metabolic-resource war, some researchers are trying to sidestep it, introducing parallel biosynthesis pathways that won’t interfere with natural processes. They’ve zeroed in on cofactors: small organic molecules that bind to enzymes and donate (reduce) or accept (oxidize) the electrons needed for enzymes to do their work. Many of these ‘redox’ reactions, in which chemical groups are added, subtracted or restructured in compounds, are energetically expensive for cells. Compounding the problem is the fact that some reactions require a relatively ‘reducing’ environment whereas others require an ‘oxidizing’ environment; making both conditions occur in the same cell is nearly impossible.
Designing synthetic redox cofactors that can be used only by synthetic enzymes could bypass the cell’s natural machinery entirely, ensuring that the two processes no longer need to compete. “It’s still using nature’s same infrastructure,” says Han Li, a chemical and biological engineer at the University of California, Irvine. These non-canonical redox cofactors (NRCs), she says, could allow synthetic biologists to create chemical reactions that are either entirely new to nature, or more efficient than those catalysed by existing enzymes.
Li’s group is among several that are engineering NRCs, and the enzymes to use them. They have already designed synthetic systems that yield more product than previous attempts. But it isn’t easy. Researchers need to find ways to efficiently produce NRCs, ensure that the cofactors and enzymes don’t harm the cells, and modify each enzyme to accept NRCs without disrupting the enzyme’s normal functions.
Scaling up NRC production to industrial levels will bring more challenges. But some researchers think that NRCs could transform chemical manufacturing, making bioproduction cheaper, easier and more viable. “Right now, biomanufacturing can’t compete with fossil-fuel based processes because of cost,” Li says. By decreasing the cost, she says, waste and emissions could decrease as well.
A universal currency
The idea of fleshing out biomanufacturing processes with a parallel set of cofactors has been around since at least the 1950s, when researchers began finding ways to synthesize alternative compounds and searching for the rare enzyme that could use them effectively. But the artificial cofactors were prohibitively expensive to make. And because natural enzymes could switch back and forth between synthetic and natural cofactors, both the cell and biomanufacturing yields suffered. Designing enzymes that specifically used a non-canonical cofactor proved an even harder problem, until advances in genetics and structural biology made protein engineering a relatively straightforward process.
Li was inspired by efforts to produce synthetic nucleotides that could be inserted into DNA, allowing genetic sequences to use six different nucleotides instead of four and drastically expanding the number of amino acids that the DNA could theoretically encode. If the same idea could be applied to cellular metabolism, she reasoned, it would expand the types of product that cells could make. “Why not do this for the universal currency of life?” she says.
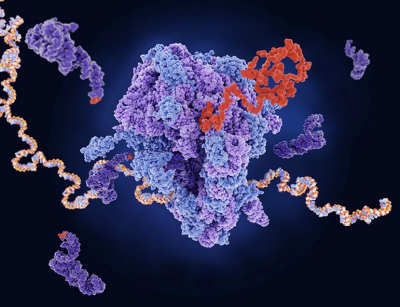
How scientists are hacking the genetic code to give proteins new powers
Cofactors are indeed a universal currency. Plant, animal and microbial cells all tend to use the same organic molecules to drive enzymatic reactions, such as ATP for energy and S-adenosyl methionine (SAM) as a source of methyl and sulfur-containing chemical groups.
One of the most common cofactors is nicotinamide adenine dinucleotide (NAD), which, with its phosphorylated form NADP, drives metabolic pathways, such as those that break down glucose into carbon dioxide and water. These cofactors exist in two configurations: a reduced form (NADH) that donates electrons and an oxidized form (NAD + ) that receives them.
A genomic analysis of the bacterium Escherichia coli estimated that the organism uses NAD in 127 enzymatic reactions and NADP in another 113 1 . NAD “is so central to life and all the ways that we understand it”, concludes Ruud Weusthuis, a microbial engineer at Wageningen University in the Netherlands.
But that centrality also means that, when a bioengineer wants to coax microbes into producing a new product — an artificial scent, for instance — they face a lot of competition for NAD. Simply providing the cells with more cofactors doesn’t help because the cells’ metabolisms just run faster. And given the choice, natural enzymes prefer to carry out easier reactions that don’t require much energy, making it hard for engineers to restrict where electrons are used. “Electrons are important, and we don’t want to waste them,” Weusthuis says.
NRCs can shift that balance of power. One of the most promising is nicotinamide mononucleotide (NMN), a subunit of NAD (see ‘Electron alternatives’). Bacterial cells naturally synthesize small amounts of NMN when producing the nucleotides that make up DNA and RNA, and adding certain enzymes to the cells can increase production.
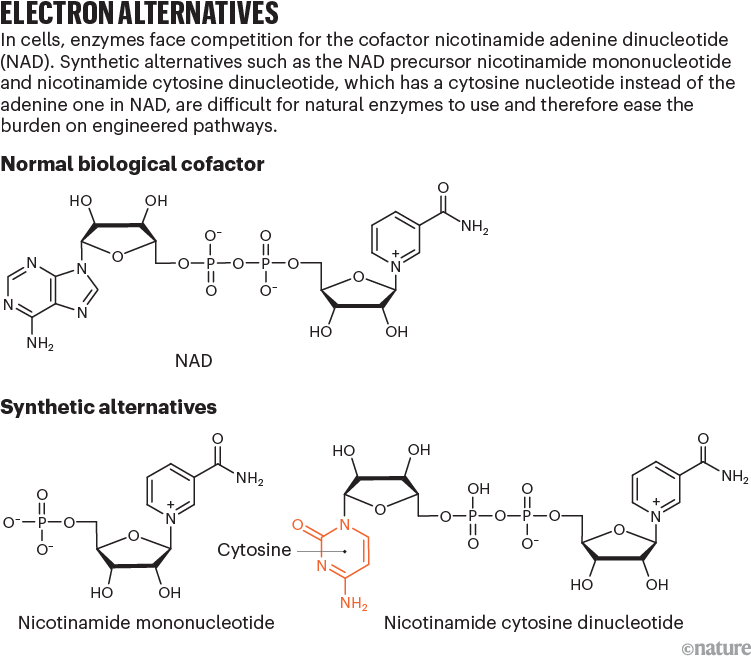
In a 2019 paper 2 , Li’s team made four changes to an enzyme called glucose dehydrogenase that made it 10 million times more likely to use NMN than NAD. By pairing this modified enzyme with another that can naturally use NMN, the group successfully induced E. coli to produce a pharmaceutical product called levodione using only artificial cofactors. The swap produced about as much levodione as the cells could produce with enzymes that use only NAD, and altering the enzyme’s structure further could increase its efficiency, the researchers say.
Li’s team has also used NMN to power more challenging syntheses, including the scent-related chemical citronellal. Citronellal is difficult to make because cells quickly convert it into other products. Engineering certain enzymes to use NMN exclusively instead of NAD allowed citronellal to accumulate in cells 3 . But, in a finding that highlights the delicate balance metabolic engineers face, Li’s group also found that when NMN + concentrations are high, cell growth suffers. Bioengineer William Black, who works with Li at the University of California, Irvine, says that the field is still working to understand how the natural enzymes affected by NMN + work, and researchers might need to optimize their bacteria on a case-by-case basis.
Other researchers are exploring different NRCs. Zongbao Kent Zhao, a bioengineer at the Dalian Institute of Chemical Physics in China, is engineering enzymes to use a cofactor called nicotinamide cytosine dinucleotide (NCD), which uses a cytosine nucleotide in place of the adenine in NAD. Unlike NMN, NCD is entirely artificial: cells do not naturally make it, although enzymes can be engineered to use it as a cofactor. Zhao hopes that this feature will prevent ‘crosstalk’ between the biosynthetic and natural metabolic pathways, which might be able to use NMN in place of NAD when levels of NMN are high, leaving less, therefore, for the desired synthesis reactions.
Zhao’s group has altered two of the enzymes in the E. coli pathway that produces malate 4 — a precursor to lactic acid — so that they use NCD instead of NAD. His eventual goal, Zhao says, is to make biofuels such as biodiesel that can be “dropped in” to combustion engines without needing to modify the engines.
Decoupling natural from biosynthetic processes could make the manufacturing process more environmentally friendly, says Pablo Iván Nikel, a biotechnologist at the Technical University of Denmark in Kongens Lyngby. “This seems to be something the industry is craving.” For chemical manufacturers using microorganisms to spin natural substances into useful chemicals, it could solve two major problems, he says: the need for fossil fuels as substrates and the production of toxic waste by-products.
Nikel’s group, for instance, has been trying to find ways to get bacteria to produce fluorinated precursors of compounds such as polytetrafluoroethylene (Teflon), which are highly toxic to manufacture. His group focuses on Pseudomonas putida , a species that thrives in toxic conditions. In 2020, the group described genetic circuits that force this tough bacterium to add fluoride to sugars and other chemicals 5 — a biological process that occurs rarely in nature. Now, the team is trying to incorporate NRCs into this process in the hope of making this difficult process more efficient, and eventually to drive reactions using carbon dioxide captured from the atmosphere. “We’re replacing this oil-dependent society with more ecofriendly production systems,” Nikel says.
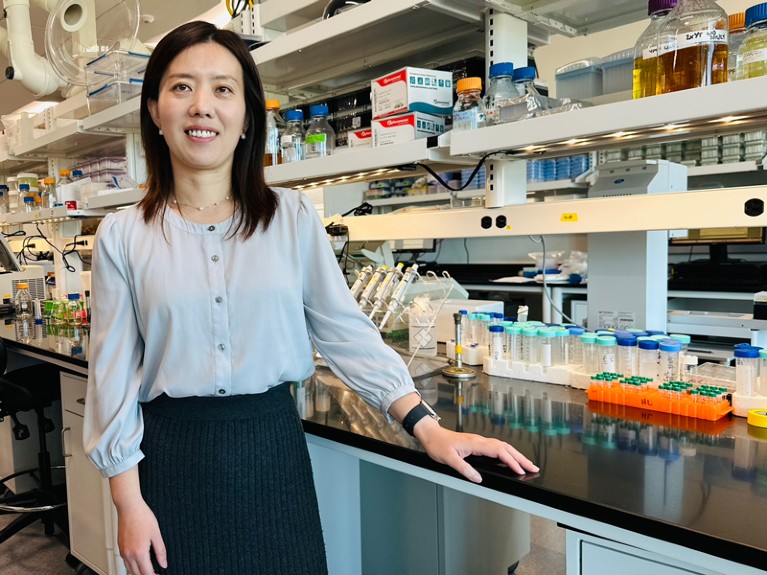
Han Li is one of several researchers investigating non-canonical redox cofactors. Credit: Natalie Tso and Cynthia Dieudonne
NAD isn’t the only redox cofactor that could be replaced with synthetic substitutes. Stephan Hammer, an organic chemist at the University of Bielefeld in Germany, and his team are developing analogues for SAM, which participates in reactions that add methyl groups or sulfur groups called sulfonium to chemical compounds.
Sulfonium reactions are rare in nature, and Hammer says that being able to control them with synthetic SAM substitutes could enable researchers to produce pharmaceutical drugs or label proteins so that they can be tracked in cells. “If you potentially have new cofactors and new reactivities, you can expand on what’s in nature,” he says. But although his group has found ways to synthesize large amounts of different SAM analogues in cells, incorporating them into synthetic pathways has been difficult because the enzymes’ activity is still low, he says.
Science fiction … in cells
Indeed, NRCs present challenges on multiple levels, specialists say. Ideally, they should work with engineered enzymes but not interact with existing enzymes, says Caroline Paul, a biological chemist at the Delft University of Technology in the Netherlands. Her group is working on NMN with Weusthuis, engineering enzymes that use it exclusively rather than interchanging it with NAD. The researchers are also finding ways to modify NMN and other cofactors with chemical tails that tailor the compounds to certain enzymes such as monooxygenases, which cells use to create signalling molecules. Researchers could eventually exploit such designs to use different NRCs at different steps of the reaction in the same cell.
For now, Paul’s team is focusing on lactic acid and ethanol production because these systems are well characterized. From there, she hopes to move onto complex chemicals such as those used in perfumes.
And that’s where NRCs could be particularly useful, says Richard van Kranenburg, a biotechnologist at Amsterdam-based biochemicals company Corbion, the world’s leading producer of industrial lactic acid. “I don’t think we could improve lactic-acid production,” he says. Incorporating NRCs and engineered enzymes would require manufacturers to set up new production pathways to yield a chemical that microorganisms naturally make very efficiently.
But decreasing costs could make biomanufacturing more viable for chemicals such as the natural plant phytoestrogens that are used in some cancer drugs. For plants, phytoestrogen synthesis is a complex, multistep and inefficient process that involves many enzymes, says Jules Beekwilder, a chemical biologist with the chemical manufacturer BASF in Geleen, the Netherlands. Companies could put these enzymes into yeast or bacteria and perhaps produce the chemical in a fermentation tank, but the many production steps mean that energy is lost throughout the process. In those cases, increased output could justify the added expenses, van Kranenburg says. “The cost of your medium doesn’t matter and maybe even the cost of the cofactor does not matter if the product is valuable enough.”
Beekwilder and van Kranenburg are industry advisers for Weusthuis’s and Paul’s research teams, although neither BASF nor Corbion fund the research. Both advisers say that they are excited about the potential of NRCs. “It may be nice to control redox conditions without affecting the physiology of the organism,” Beekwilder says. But he adds that scaling them up to meet consumer demand presents its own set of challenges, such as maintaining the varying levels of oxygen needed for different enzymatic reactions. And although researchers often know what each enzyme does, they might not understand the biophysics well enough to be able to modify the enzyme to accept an NRC. “It’s not so that you can press the button on the computer and it’s done,” he says.
That said, advances in artificial intelligence (AI) are making enzyme engineering much easier than it was just a few years ago. “The idea that we have to crystallize a protein is ending,” Pfleger says, referring to the classic structural-biology technique of X-ray crystallography. “The ability to give proteins new functions or design from scratch is accelerating like crazy.” At the same time, AI tools such as AlphaFold and RoseTTAFold are learning how to decode enzymes’ structure from their sequence and could predict how specific modifications — a new fold that allows the enzyme to accept NMN instead of NAD, for instance — would affect its function.
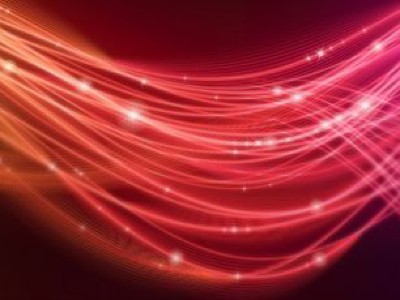
NatureTech hub
Li says that she is “very excited” about the possibilities of AI and hopes one day to accumulate enough data to work out which modifications are needed to make any enzyme work with NRCs, and to start automating the process for creating them. Her group has already begun working out design principles that would allow enzymes to switch their preference from NAD to NMN.
Researchers might even be able to modify certain NRC-powered enzymes to be more powerful than their natural counterparts, or to work at different acidities, temperatures or oxygen levels from those that are normally required by natural enzymes. If the enzymes are versatile enough, Li estimates that NMN-powered reactions could end up being significantly cheaper than those that use NAD, owing to the increased efficiency and, potentially, the lower cost of producing NMN compared with NAD.
Still, researchers would prefer to be as efficient as possible. In nature, biosynthetic redox reactions are coupled with secondary reactions that recycle NADH to NAD + , or vice versa, so that the cell can keep the biosynthesis going. Research groups including Li’s are designing ‘recycling’ enzymes that can switch NMN + to and from NMNH, creating oxidative or reductive conditions in the cell that are independent of NAD. Ultimately, Li hopes to find or develop a universal recycling enzyme or electrode that can switch any NRC back to its oxidized or reduced forms, depending on what the artificial biosynthetic pathway needs.
Not that every enzyme in a complex synthesis pathway would need to depend on an NRC, Hammer notes; the engineered enzymes could be reserved for particularly inefficient bottlenecks, or to make minor modifications to a compound that the cell has already made.
As the roster of NRCs expands, says Weusthuis, the biosynthetic possibilities are endless. “This is science fiction, not in space but in cells.”
Nature 628 , 224-226 (2024)
doi: https://doi.org/10.1038/d41586-024-00907-x
Monk, J. et al. Nature Biotechnol. 35 , 904–908 (2017).
Article PubMed Google Scholar
Black, W. B. et al. Nature Chem. Biol. 16 , 87–94 (2020).
Richardson, K. N., Black, W. B. & Li, H. ACS Catal . 10 , 8898–8903 (2020).
Guo, X. et al. Angew. Chem. Int. Ed. Engl. 59 , 3143–3146 (2020).
Calero, P. et al. Nature Commun. 11 , 5045 (2020).
Download references
Related Articles
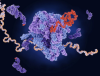
- Biotechnology
- Microbiology
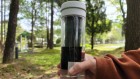
This water bottle purifies your drink with energy from your steps
Research Highlight 17 APR 24
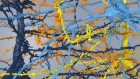
A milestone map of mouse-brain connectivity reveals challenging new terrain for scientists
Technology Feature 15 APR 24
How to break big tech’s stranglehold on AI in academia
Correspondence 09 APR 24
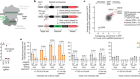
Improving prime editing with an endogenous small RNA-binding protein
Article 03 APR 24
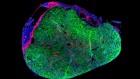
‘Mini liver’ will grow in person’s own lymph node in bold new trial
News 02 APR 24
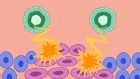
Video: Cancer-busting vaccines
Outline 27 MAR 24
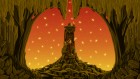
Exploring the lung microbiome’s role in disease
Outlook 17 APR 24
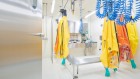
Deadly diseases and inflatable suits: how I found my niche in virology research
Spotlight 17 APR 24
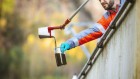
What toilets can reveal about COVID, cancer and other health threats
News Feature 17 APR 24
2024 Recruitment notice Shenzhen Institute of Synthetic Biology: Shenzhen, China
The wide-ranging expertise drawing from technical, engineering or science professions...
Shenzhen,China
Shenzhen Institute of Synthetic Biology
Recruitment of Global Talent at the Institute of Zoology, Chinese Academy of Sciences (IOZ, CAS)
The Institute of Zoology (IOZ), Chinese Academy of Sciences (CAS), is seeking global talents around the world.
Beijing, China
Institute of Zoology, Chinese Academy of Sciences (IOZ, CAS)
Research Associate - Brain Cancer
Houston, Texas (US)
Baylor College of Medicine (BCM)
Senior Manager, Animal Care
Research associate - genomics.
Sign up for the Nature Briefing newsletter — what matters in science, free to your inbox daily.
Quick links
- Explore articles by subject
- Guide to authors
- Editorial policies
share this!
April 22, 2024
This article has been reviewed according to Science X's editorial process and policies . Editors have highlighted the following attributes while ensuring the content's credibility:
fact-checked
peer-reviewed publication
trusted source
AI tool creates 'synthetic' images of cells for enhanced microscopy analysis
by University of California - Santa Cruz
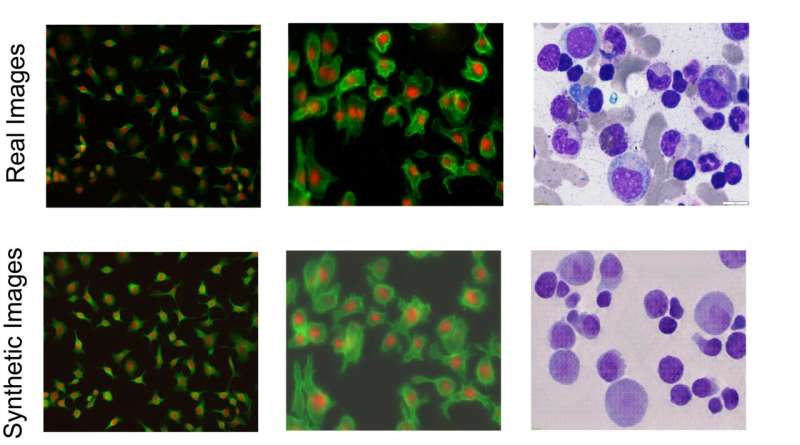
Observing individual cells through microscopes can reveal a range of important cell biological phenomena that frequently play a role in human diseases, but the process of distinguishing single cells from each other and their background is extremely time-consuming—and a task that is well-suited for AI assistance.
AI models learn how to carry out such tasks by using a set of data that are annotated by humans, but the process of distinguishing cells from their background, called "single-cell segmentation," is both time-consuming and laborious. As a result, there is a limited amount of annotated data to use in AI training sets. UC Santa Cruz researchers have developed a method to solve this by building a microscopy image generation AI model to create realistic images of single cells, which are then used as " synthetic data " to train an AI model to better carry out single cell-segmentation.
The new software is described in a new paper published in the journal iScience . The project was led by Assistant Professor of Biomolecular Engineering Ali Shariati and his graduate student Abolfazl Zargari. The model, called cGAN-Seg, is freely available on GitHub .
"The images that come out of our model are ready to be used to train segmentation models," Shariati said. "In a sense we are doing microscopy without a microscope, in that we are able to generate images that are very close to real images of cells in terms of the morphological details of the single cell. The beauty of it is that when they come out of the model, they are already annotated and labeled. The images show a ton of similarities to real images, which then allows us to generate new scenarios that have not been seen by our model during the training."
Images of individual cells seen through a microscope can help scientists learn about cell behavior and dynamics over time, improve disease detection, and find new medicines. Subcellular details such as texture can help researchers answer important questions, like whether a cell is cancerous or not.
Manually finding and labeling the boundaries of cells from their background is extremely difficult, however, especially in tissue samples where there are many cells in an image. It could take researchers several days to manually perform cell segmentation on just 100 microscopy images.
Deep learning can speed up this process, but an initial data set of annotated images is needed to train the models—at least thousands of images are needed as a baseline to train an accurate deep learning model. Even if the researchers can find and annotate 1,000 images, those images may not contain the variation of features that appear across different experimental conditions.
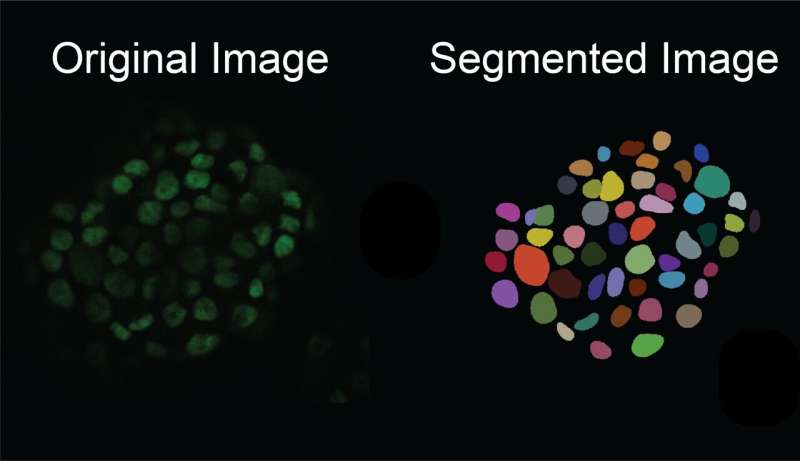
"You want to show your deep learning model works across different samples with different cell types and different image qualities," Zargari said. "For example, if you train your model with high-quality images, it's not going to be able to segment the low-quality cell images. We can rarely find such a good data set in the microscopy field."
To address this issue, the researchers created an image-to-image generative AI model that takes a limited set of annotated, labeled cell images and generates more, introducing more intricate and varied subcellular features and structures to create a diverse set of "synthetic" images. Notably, they can generate annotated images with a high density of cells, which are especially difficult to annotate by hand and are especially relevant for studying tissues. This technique works to process and generate images of different cell types as well as different imaging modalities, such as those taken using fluorescence or histological staining.
Zargari, who led the development of the generative model, employed a commonly used AI algorithm called a "cycle generative adversarial network" for creating realistic images. The generative model is enhanced with so-called "augmentation functions" and a "style injecting network," which helps the generator to create a wide variety of high-quality synthetic images that show different possibilities for what the cells could look like. To the researchers' knowledge, this is the first time style injecting techniques have been used in this context.
Then, this diverse set of synthetic images created by the generator is used to train a model to accurately carry out cell segmentation on new, real images taken during experiments.
"Using a limited data set, we can train a good generative model. Using that generative model, we are able to generate a more diverse and larger set of annotated, synthetic images. Using the generated synthetic images we can train a good segmentation model—that is the main idea," Zagari said.
The researchers compared the results of their model using synthetic training data to more traditional methods of training AI to carry out cell segmentation across different types of cells. They found that their model produces significantly improved segmentation compared to models trained with conventional, limited training data. This confirms to the researchers that providing a more diverse dataset during training of the segmentation model improves performance.
Through these enhanced segmentation capabilities, the researchers will be able to better detect cells and study variability between individual cells , especially among stem cells. In the future, the researchers hope to use the technology they have developed to move beyond still images to generate videos, which can help them pinpoint which factors influence the fate of a cell early in its life and predict their future.
"We are generating synthetic images that can also be turned into a time lapse movie, where we can generate the unseen future of cells," Shariati said. "With that, we want to see if we are able to predict the future states of a cell, like if the cell is going to grow, migrate, differentiate or divide."
Journal information: iScience
Provided by University of California - Santa Cruz
Explore further
Feedback to editors

Liquid droplets shape how cells respond to change, shows study
3 hours ago

Rice bran nanoparticles show promise as affordable and targeted anticancer agent

Advance in forensic fingerprint research provides new hope for cold cases

How spicy does mustard get depending on the soil?

Electron videography captures moving dance between proteins and lipids
4 hours ago

New findings shed light on how bella moths use poison to attract mates

Announcing the birth of QUIONE, a unique analog quantum processor

World's oases threatened by desertification, even as humans expand them
5 hours ago

NASA's Voyager 1 resumes sending engineering updates to Earth

Researchers break down pizza box recycling challenges, one slice at a time
Relevant physicsforums posts, the cass report (uk).
2 hours ago
Major Evolution in Action
If theres a 15% probability each month of getting a woman pregnant....
Apr 19, 2024
Can four legged animals drink from beneath their feet?
Apr 15, 2024
Mold in Plastic Water Bottles? What does it eat?
Apr 14, 2024
Dolphins don't breathe through their esophagus
More from Biology and Medical
Related Stories

Novel deep learning-based software detects and tracks individual cells with high precision
Jun 14, 2023

Images of simulated cities help artificial intelligence to understand real streetscapes
Sep 14, 2023

Microscopy image segmentation via point and shape regularized data synthesis
Oct 3, 2023

DeepWMH: A deep learning tool for accurate white matter hyperintensity segmentation

Towards smarter agriculture: Automatic identification of crop heads with artificial intelligence
Apr 20, 2023

Machine learning radically reduces workload of cell counting for disease diagnosis
May 20, 2022
Recommended for you

Kissing bugs, vector for Chagas disease, successfully gene edited for first time
7 hours ago

New super-resolution microscopy approach visualizes internal cell structures and clusters via selective plane activation
8 hours ago

A small factor makes a big impact on genome editing
9 hours ago

The enemy within: How pathogens spread unrecognized in the body

Pressure in the womb may influence facial development
10 hours ago
Let us know if there is a problem with our content
Use this form if you have come across a typo, inaccuracy or would like to send an edit request for the content on this page. For general inquiries, please use our contact form . For general feedback, use the public comments section below (please adhere to guidelines ).
Please select the most appropriate category to facilitate processing of your request
Thank you for taking time to provide your feedback to the editors.
Your feedback is important to us. However, we do not guarantee individual replies due to the high volume of messages.
E-mail the story
Your email address is used only to let the recipient know who sent the email. Neither your address nor the recipient's address will be used for any other purpose. The information you enter will appear in your e-mail message and is not retained by Phys.org in any form.
Newsletter sign up
Get weekly and/or daily updates delivered to your inbox. You can unsubscribe at any time and we'll never share your details to third parties.
More information Privacy policy
Donate and enjoy an ad-free experience
We keep our content available to everyone. Consider supporting Science X's mission by getting a premium account.
E-mail newsletter
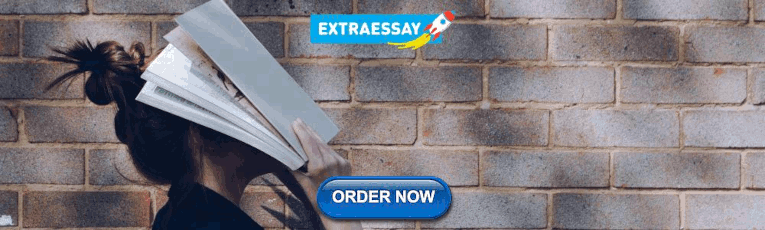
IMAGES
VIDEO
COMMENTS
The Systems, Synthetic, and Quantitative Biology PhD Program aims to explain how higher level properties of complex biological systems arise from the interactions among their parts. This field requires a fusion of concepts from many disciplines, including biology, computer science, applied mathematics, physics and engineering. ...
You can find degree program-specific admissions requirements below and access additional guidance on applying from the Systems, Synthetic, and Quantitative Biology PhD Program. Academic Background Applicants typically have a background in biology, physics, chemistry, computer science, engineering, or mathematics and work to forge a new approach ...
Synthetic Biology (SB) explores new forms of engagement with life and living systems. From molecular to ecological, cultural to political, SB is about understanding life's fundamental mysteries and translating knowledge to imagine a biotic civilization that flourishes in partnership with Earth. The Stanford Synthetic Biology community enables ...
A graduate certificate in synthetic biology will provide official recognition that students have received a multifaceted education in synthetic biology through Northwestern's unique approach to synthetic biology training and prepare graduates to enter the biotechnology workforce. The Synthetic Biology minor is intended for MS students.
The SSPB Graduate Program, housed in the Rice Synthetic Biology Institute (RSBI), is designed to provide students the knowledge and tools that they will need to solve hard research questions and to apply their discoveries to the development of biotechnologies that transform agriculture, electronics, energy, the environment, information storage, medicine, and manufacturing.
Synthetic Biology. Synthetic Biology is an emerging field of research where researchers construct new biological systems and redesign existing biological systems. Faculty in BE are studying synthetic biology from the foundational level of developing a programming language for cells to the applied level of engineering viruses to secrete enzymes ...
George leads Synthetic Biology at the Wyss Institute, where he oversees the directed evolution of molecules, polymers, and whole genomes to create new tools with applications in regenerative medicine and bio-production of chemicals. Among his recent work at the Wyss is development of a technology for synthesizing whole genes, and engineering ...
The Systems, Synthetic, and Quantitative Biology PhD Program. aims to explain how higher level properties of complex biological systems arise from the interactions among their parts. This field requires a fusion of concepts from many disciplines, including biology, computer science, applied mathematics, physics and engineering. Students with ...
Synthetic Biology (3) Apply Synthetic Biology filter; Systems Biology (4) Apply Systems Biology filter; Technology Development (64) Apply Technology Development filter; Organism. ... PhD Program in Biological & Biomedical Sciences Harvard Medical School Tosteson Medical Education Center, Suite 435 Boston, MA 02115
Graduate students can find their way to a synthetic biology research lab through several degree programs. The main avenue as a graduate student to participate in synthetic biology at Northwestern is to explore the PhD and master's degrees in more than 70 disciplines offered by The Graduate School (TGS). Find your path at Northwestern. 1.
The SynBAS program consists of 5 components: Courses in Synthetic Biology. The synthetic biology core curriculum consists of a required case-study course on deconstructing biological function across scales. Elective courses along two different scales and chosen by the students provide rigorous training in the fundamentals of physics, chemistry ...
Graduate students within the CPSB program are exposed to a variety of aspects of synthetic biology that aid them in designing and engineering biological systems useful in research areas as versatile as signal transduction, virology, metabolism, medicinal chemistry, structural biology, human disease modeling, and many others.
Biography: Prof. Weiss is one of the pioneers of synthetic biology. He has been engaged in synthetic biology research since 1996 when he was a graduate student at MIT and where he helped set up a wet-lab in the Electrical Engineering and Computer Science Department. After completion of his PhD, Weiss joined the faculty at Princeton University ...
Managing her own synthetic biology project helped graduate student Jesse Tordoff overcome imposter syndrome and hit her stride. Read more about Hacking life inside and outside the laboratory; ... MIT Computational & Systems Biology PhD Program (CSB) Massachusetts Institute of Technology 77 Massachusetts Avenue Bldg 68, Room 120C
Synthetic biology seeks to build parts, devices, and systems from biological components. The goals of these efforts can include using microorganisms to synthesize materials of medical or industrial value, and even to repurpose bacteria to fight disease. Efforts within systems biology focus on collection, curation, and analysis of large data ...
The following requirements are in addition to, or further elaborate upon, those requirements outlined in The Graduate School Policy Guide. In addition to meeting the PhD/MS requirements of their chosen departments, students will be required to complete the coursework described below: . CHEM ENG 376 - Principles of Synthetic Biology
Sustainable bio-hydrogen production via engineered microbial metabolism (4-year fully funded PhD studentship with Dstl) University of Edinburgh School of Biological Sciences. Research Group: Institute of Quantitative Biology, Biochemistry and Biotechnology. Background and the project. In 2022, carbon emissions from the chemical industry peaked ...
Programme description. The first-of-its-kind dual award PhD programme in Synthetic and Systems Biology brings together two globally-renowned institutions at the forefront of research in this area: the Manchester Institute of Biotechnology (MIB) at The University of Manchester, and the Center for Synthetic and Systems Biology (CSSB) at Tsinghua ...
MD/PhD students Andrew Anzalone and Sakellarios Zairis combined approaches based in chemical biology, synthetic biology, and computational biology to develop a new method for protein engineering. The ribosome is a reliable machine in the cell, precisely translating the nucleotide code carried by messenger RNAs (mRNAs) into the polypeptide ...
Synthetic Biology. Rice University has over 20 Synthetic Biology research groups, which share the goal of overcoming central challenges in engineering biology. These labs are pioneering new tools, technologies, and theories to transform our ability to predictably design biological systems. This includes the development of programmable ...
Year of entry: 2024. The standard academic entry requirement for this PhD is an upper second-class (2:1) honours degree in a discipline directly relevant to the PhD (or international equivalent) OR any upper-second class (2:1) honours degree and a Master's degree at merit in a discipline directly relevant to the PhD (or international ...
Synthetic biology is a discipline that uses biological components as building blocks in the design of new systems to solve crucial global challenges. ... (SynBAS), a program focused on convergent synthetic biology training for graduate students, another first-of-its-kind synthetic biology-focused program.
In addition to these first-rate employee benefits, you will receive a fully funded PhD position and you will be offered a course program tailored to your needs and the research team. The gross salary for the first year is € 2.770 - per month rising to € 3.539,- in the fourth year in according to the Collective Labour Agreements for Dutch ...
Synthetic small-molecule cofactors could reduce the cost of biomanufacturing. Credit: Alamy ... until advances in genetics and structural biology made protein engineering a relatively ...
PhD candidate Seden Bedir moved from Turkey to the U.S. to study at UMass Chan Medical School. She aims to engineer RNA sequences that don't exist in nature in order to enable mRNA-based therapeutics beyond vaccines. ... "I love all science in general—physics, chemistry, biology—but the aspect of synthetic biology intrigued me. The fact ...
Synthetic biology has recently grown out of its infancy as primarily DNA- and protein-centred research. However, proteins remain the dominant biobrick. It should then come as no surprise that more and more exciting and innovative research using RNA is being reported. These highlights reveal the enormous potential of synthetic RNA biology ...
ACS Synthetic Biology has been certified as a transformative journal by cOAlition S, committing to a transition to 100% open access in the future. If your research funder has signed Plan S, your open access charges may be covered by your funder through December 31, 2024.
The researchers compared the results of their model using synthetic training data to more traditional methods of training AI to carry out cell segmentation across different types of cells.